Dr DYPIEMR AKURDI Unit 5 Thermal Engineering Mr
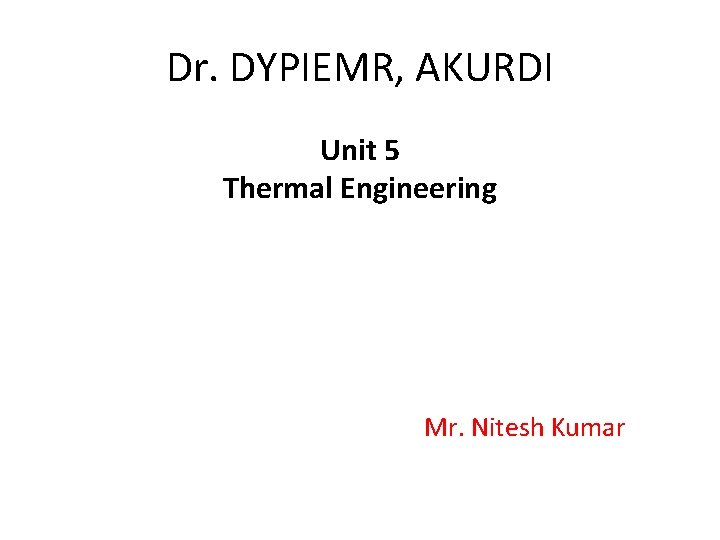
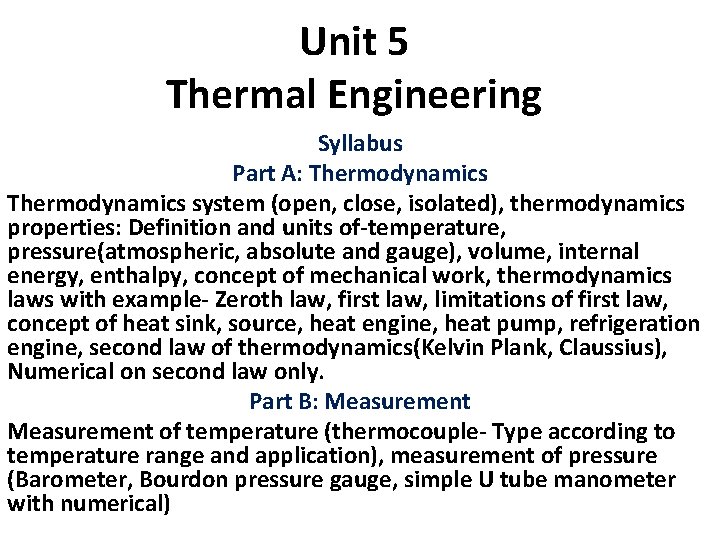
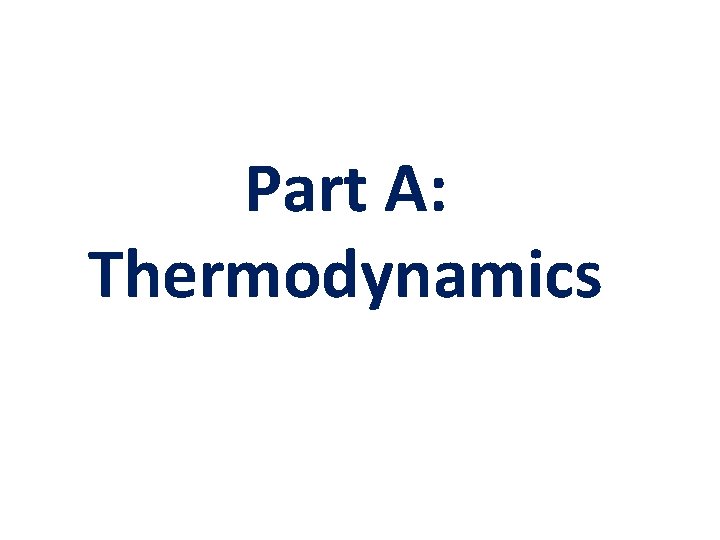
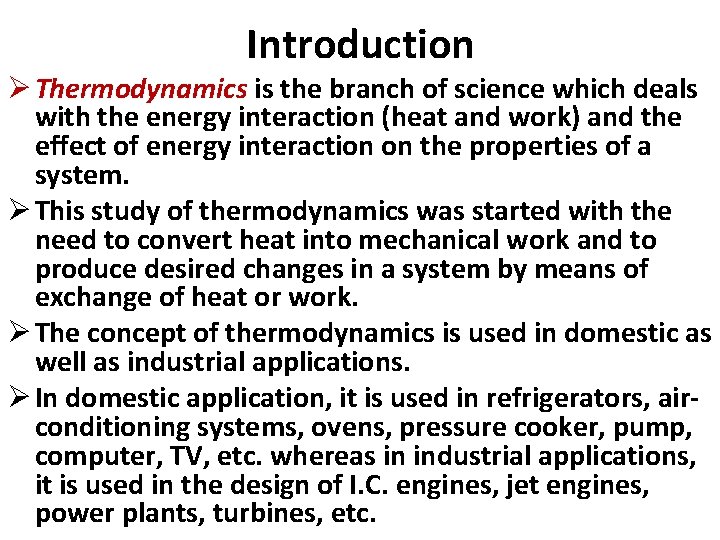
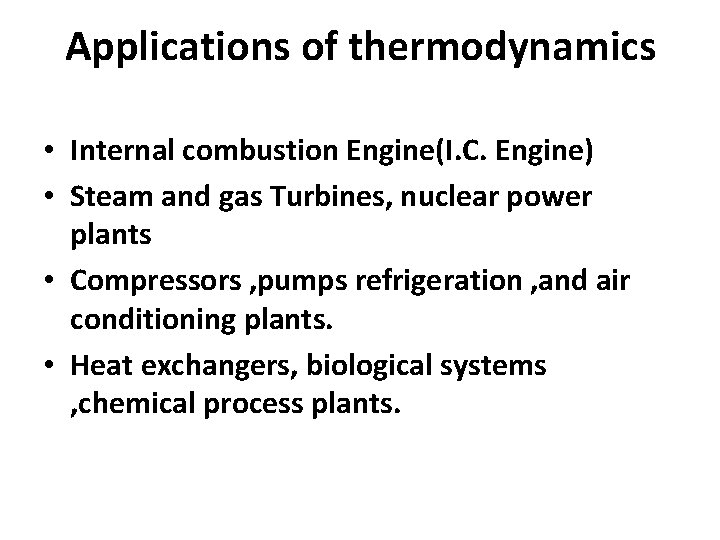
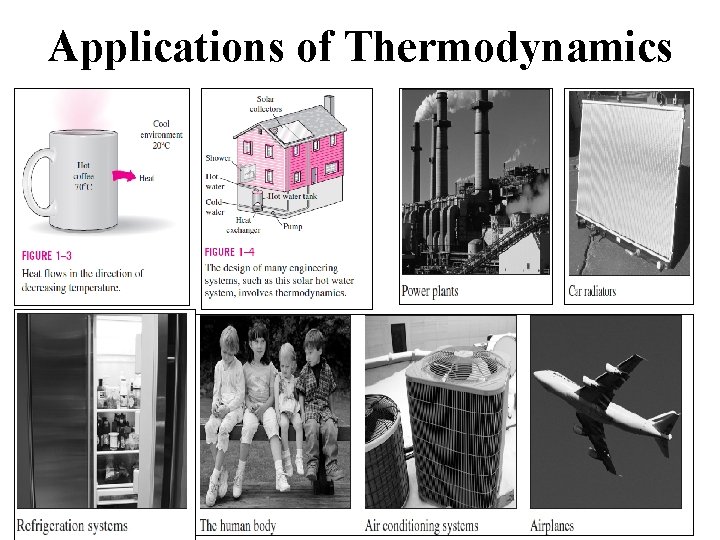
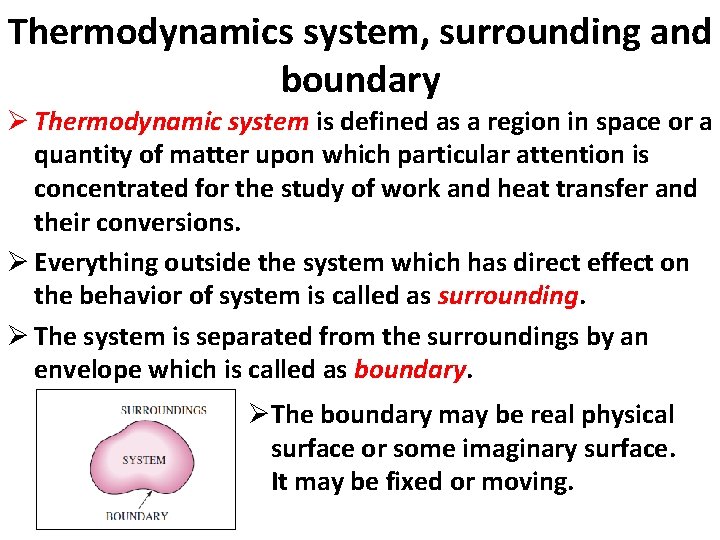
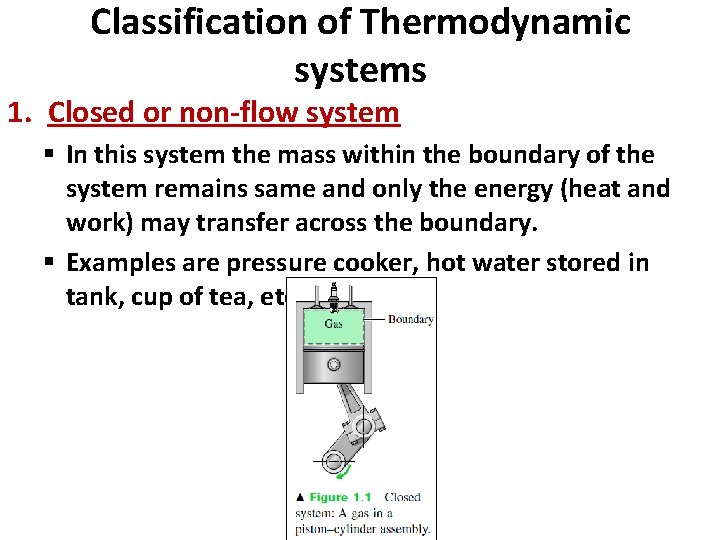
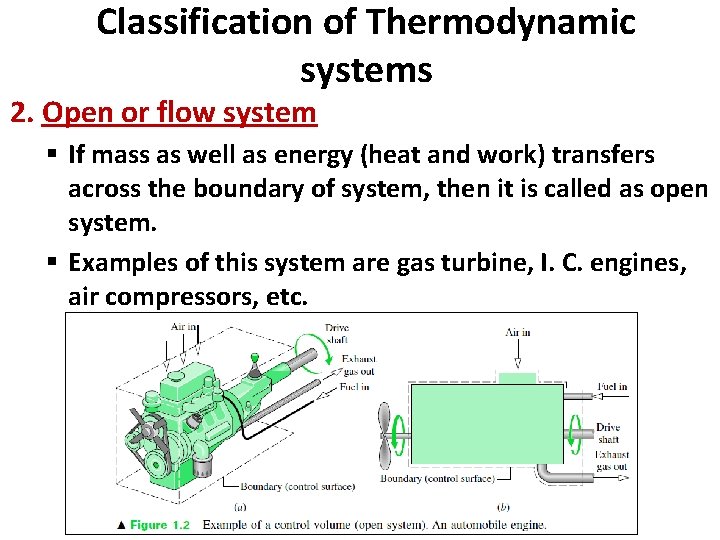
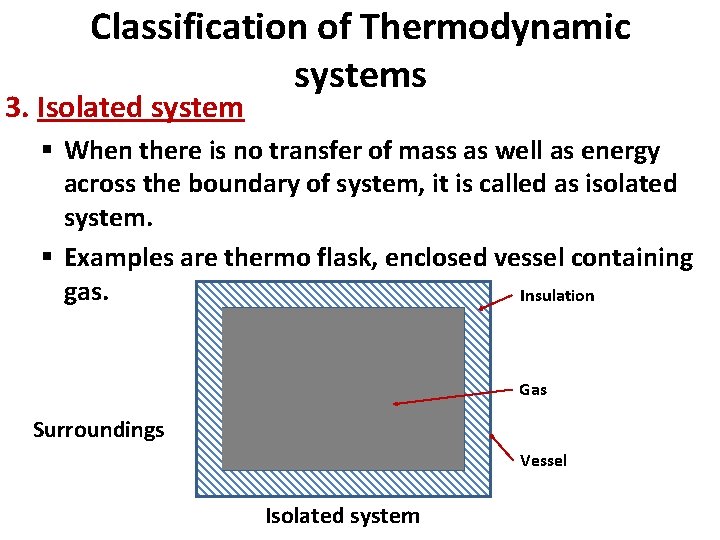
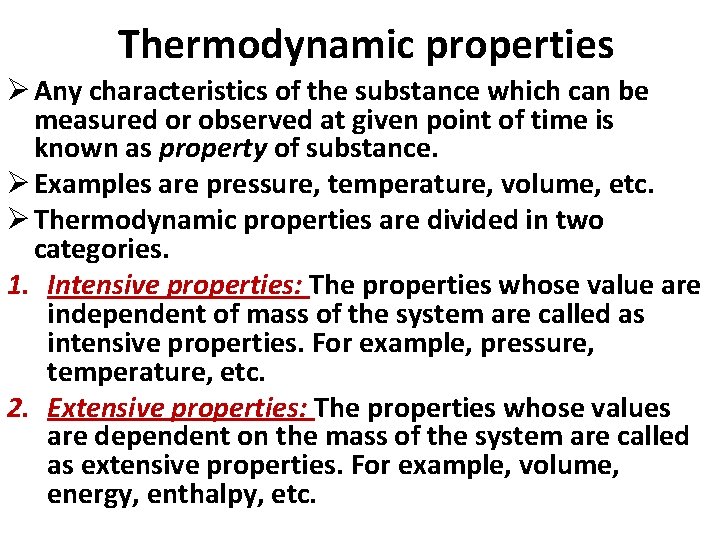
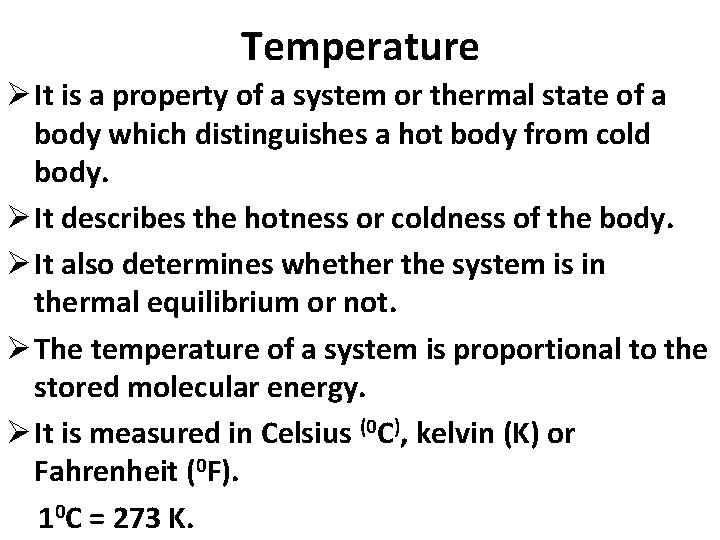
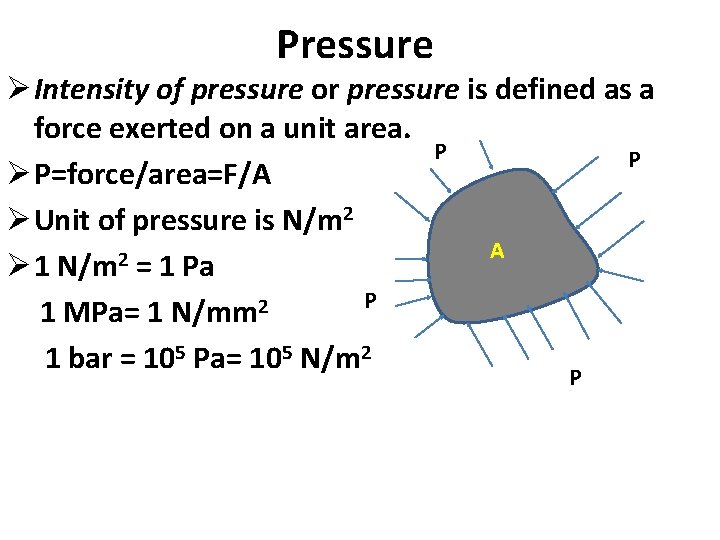
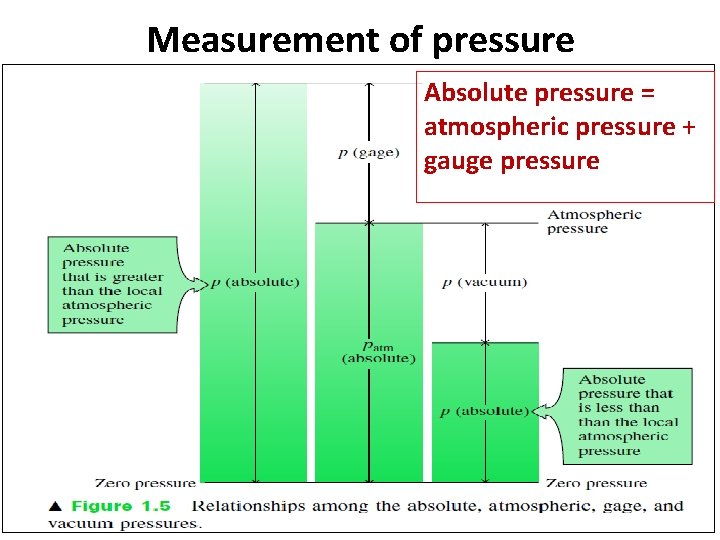
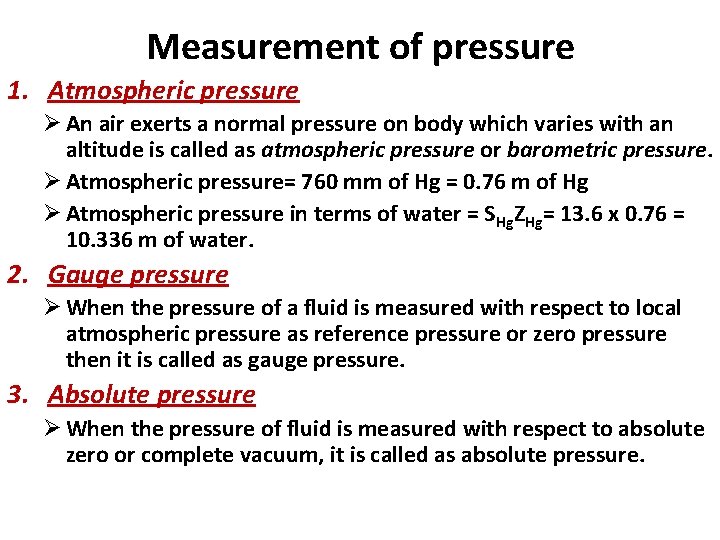
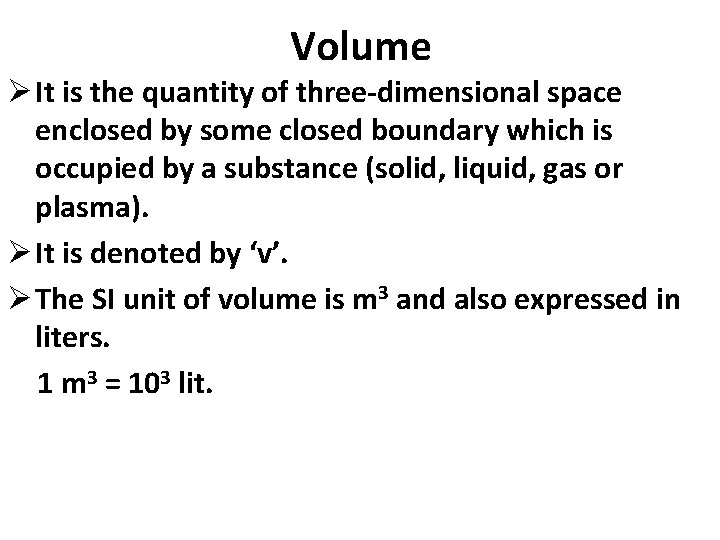
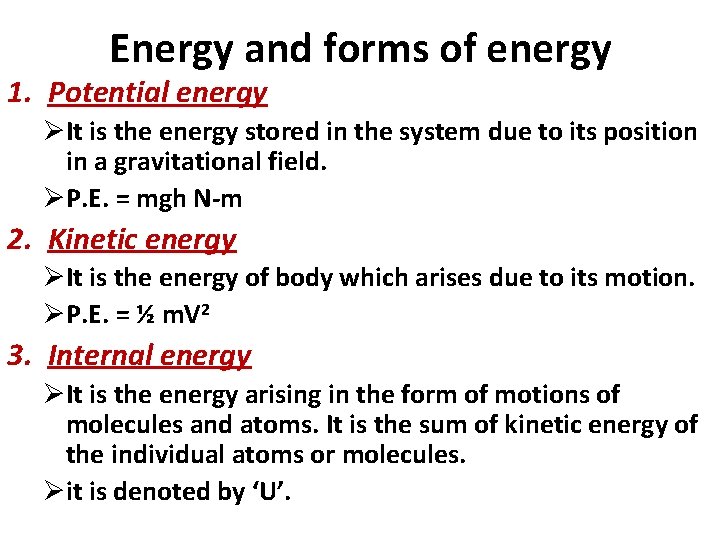
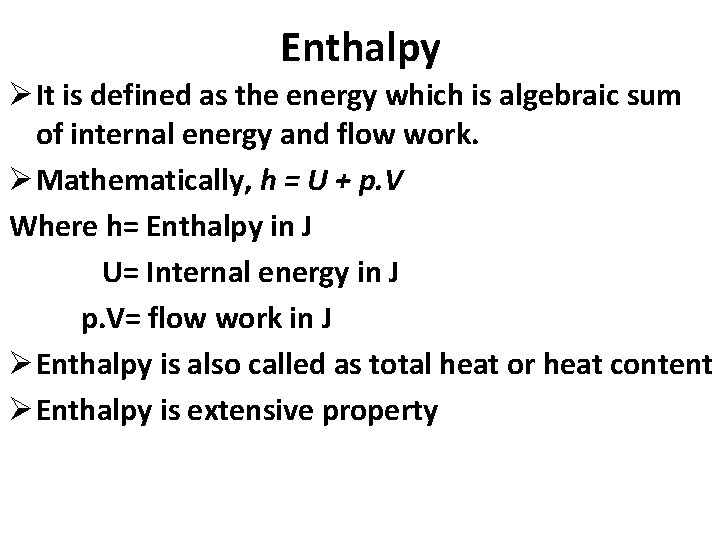
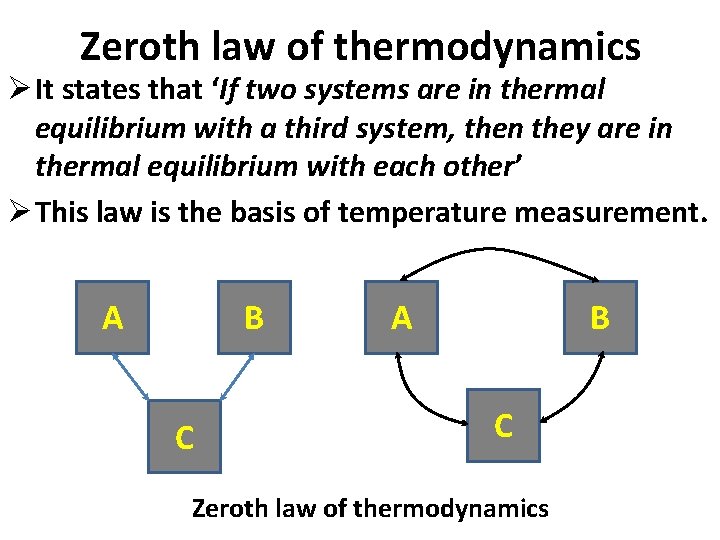
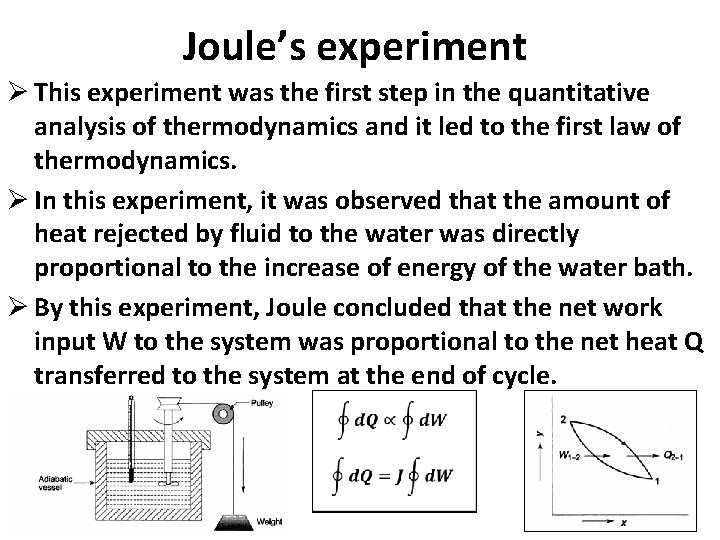
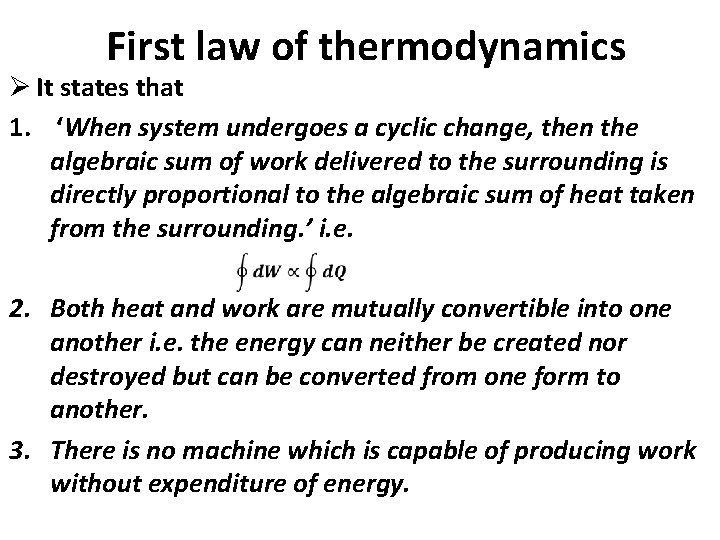
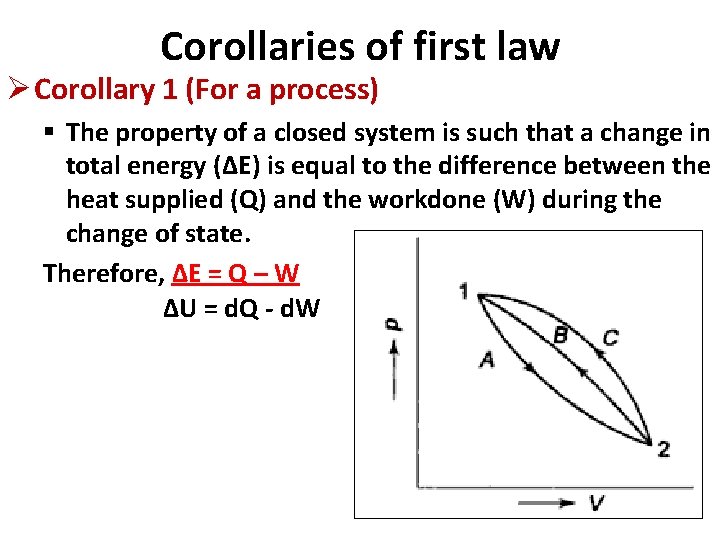
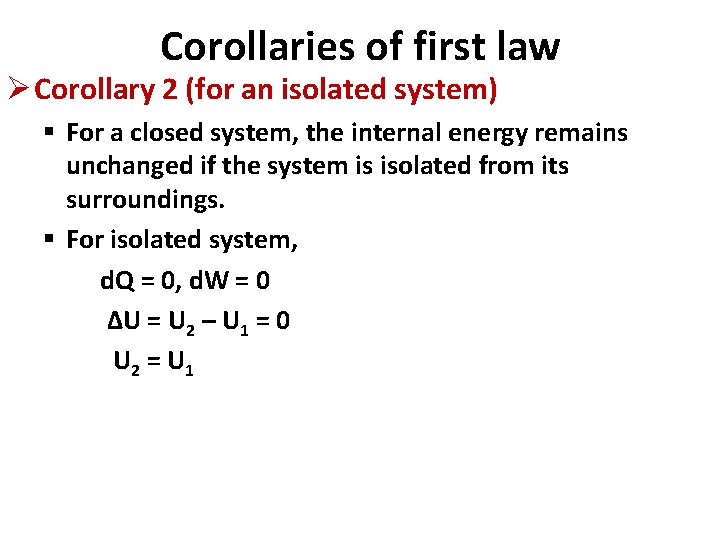
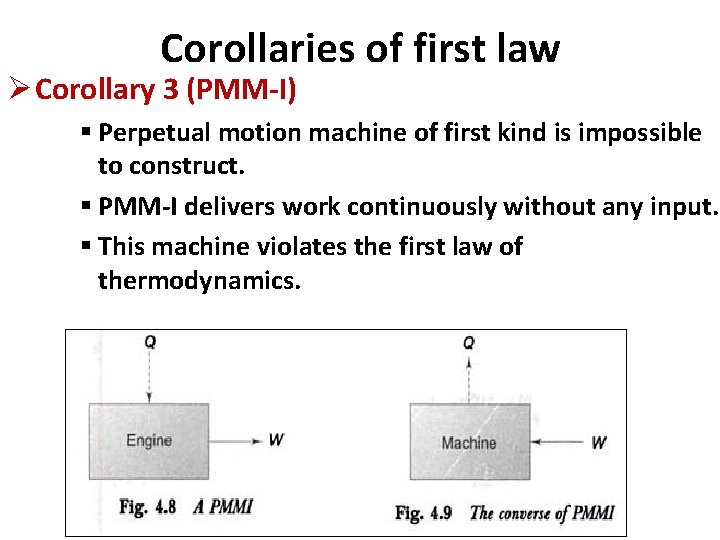
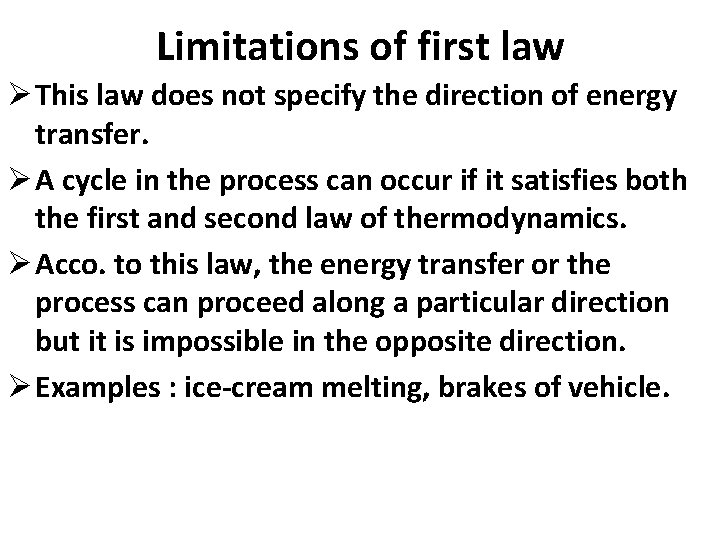
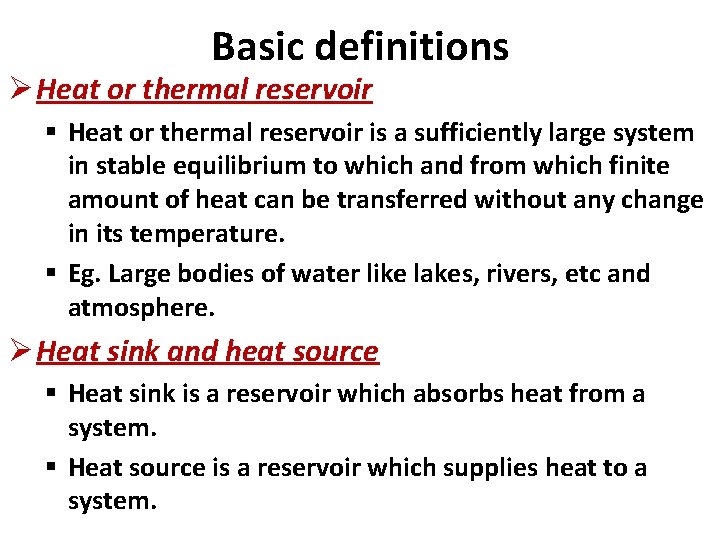
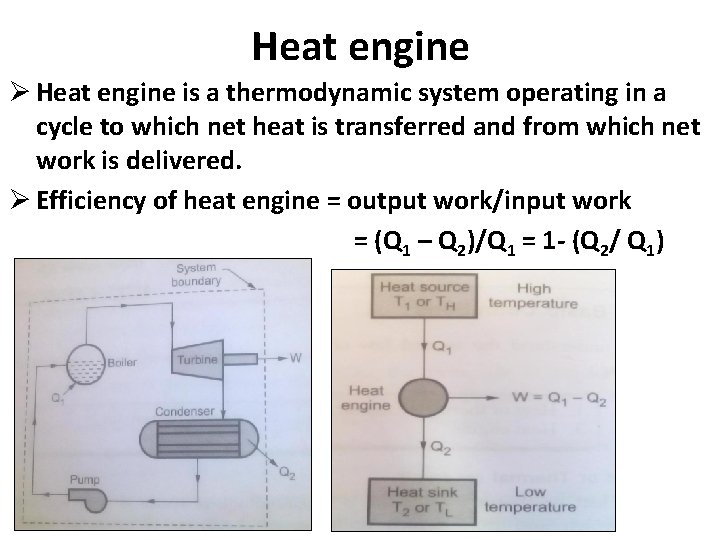
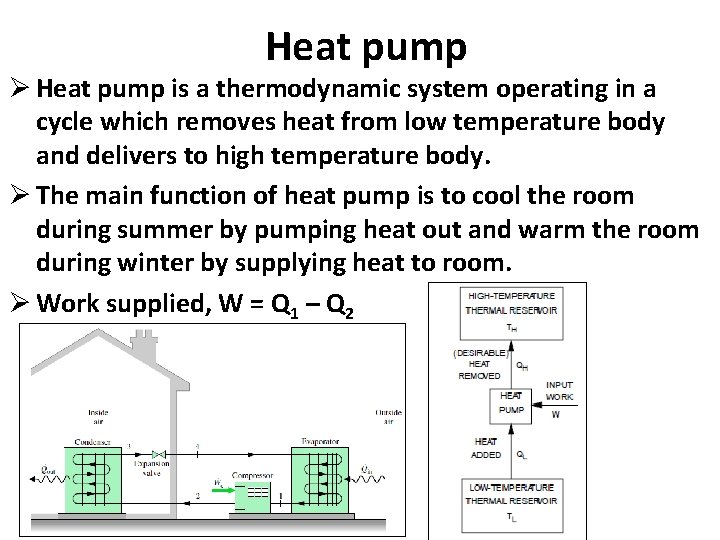
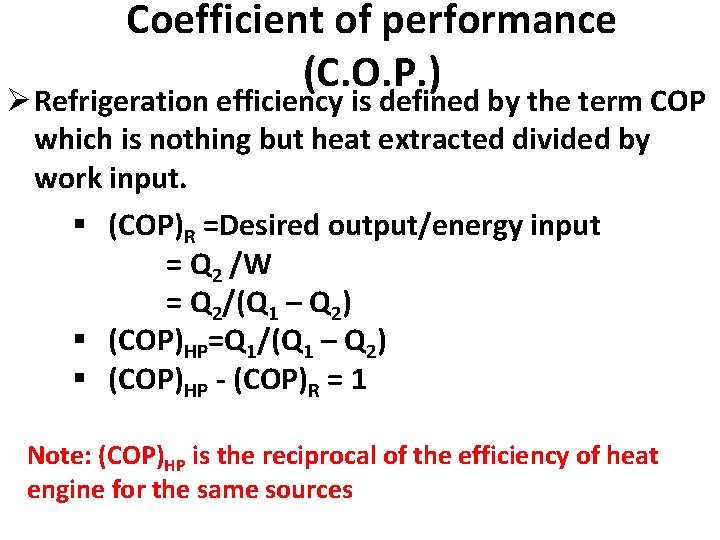
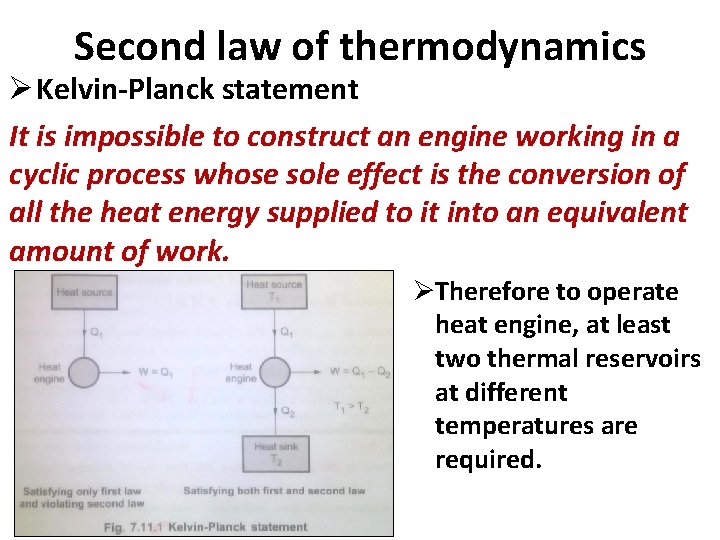
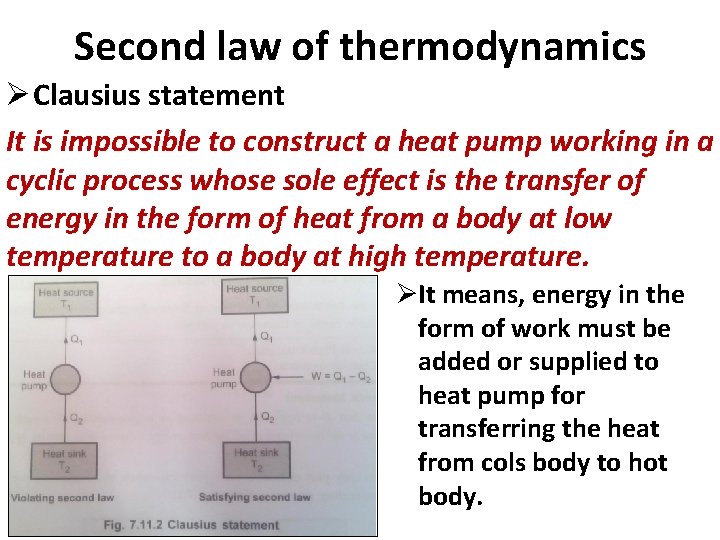
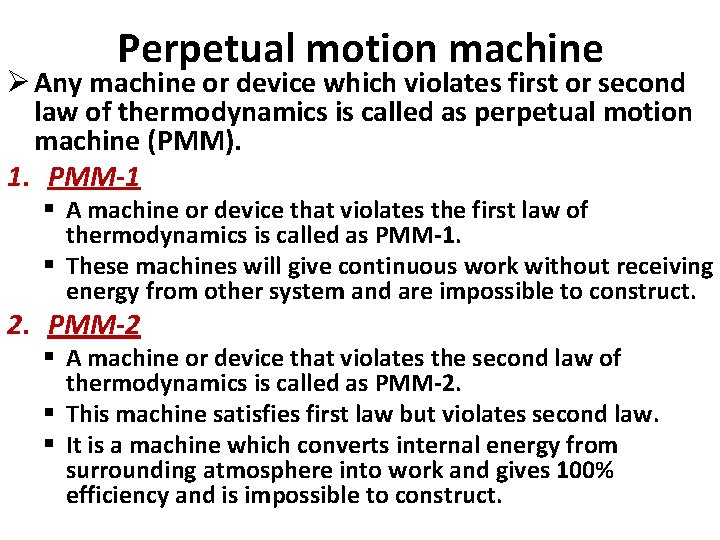
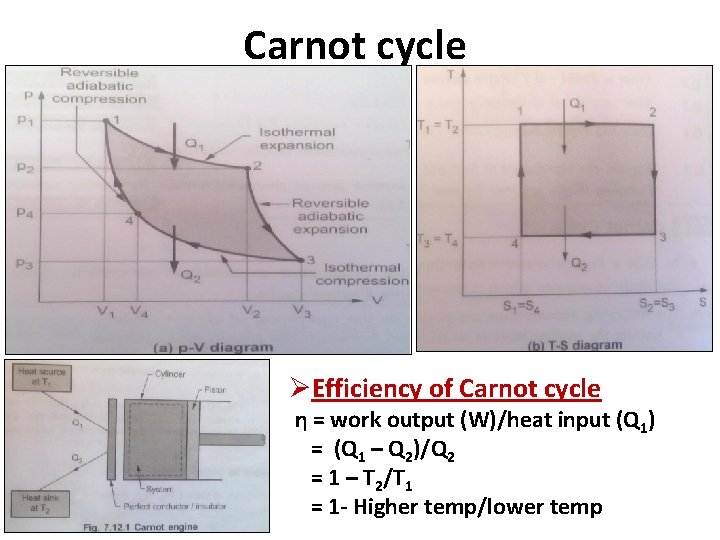
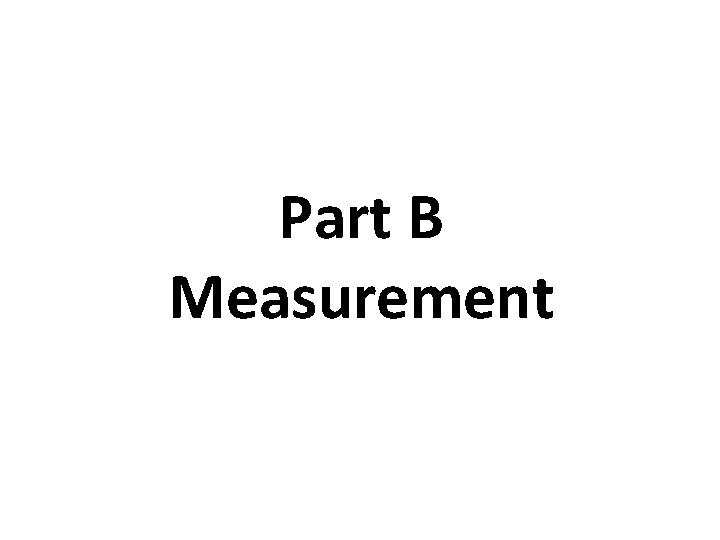
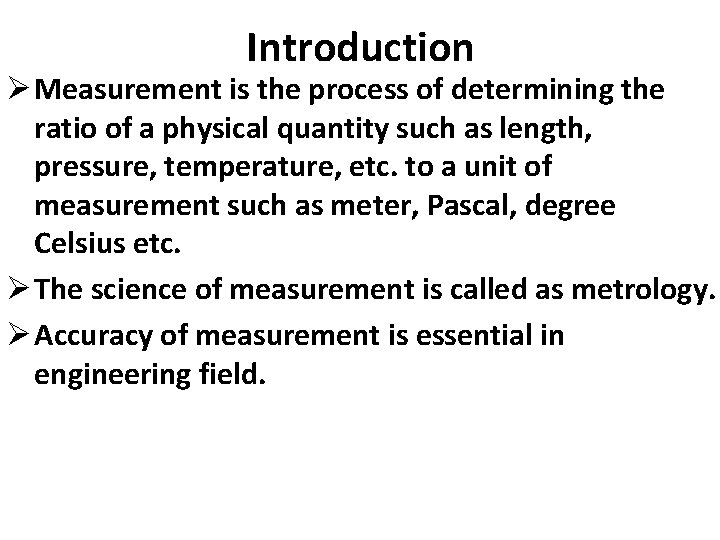
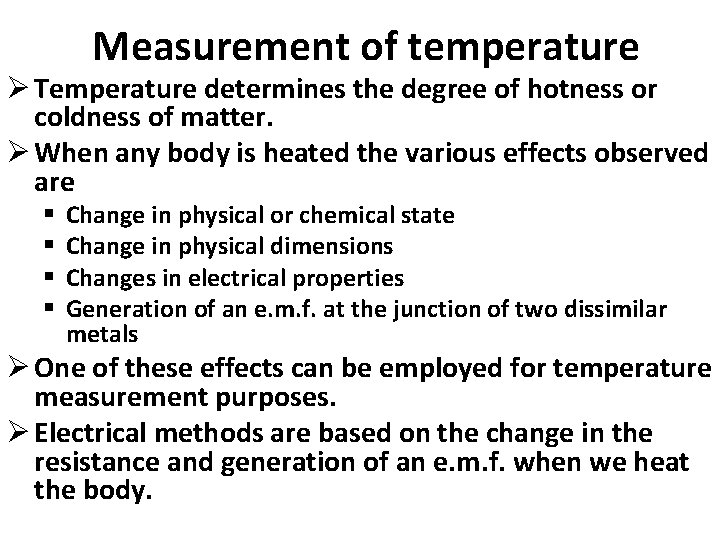
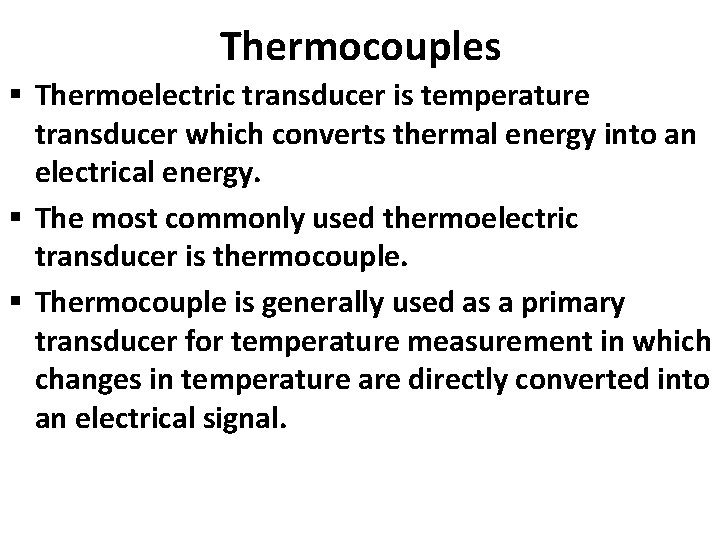
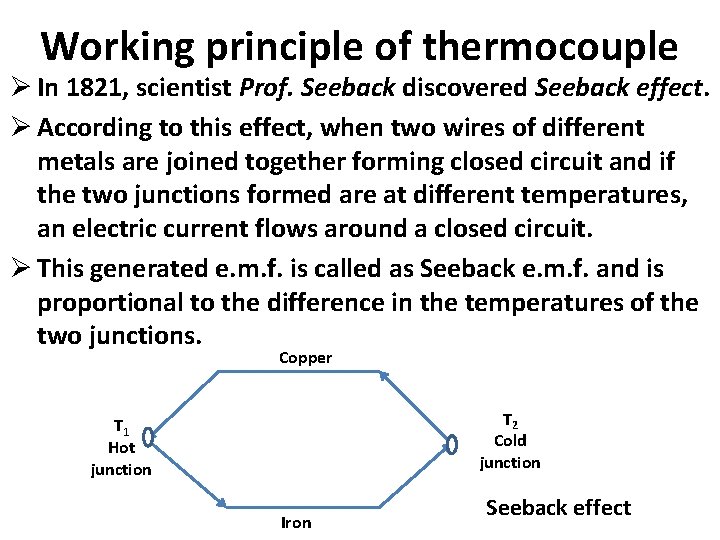
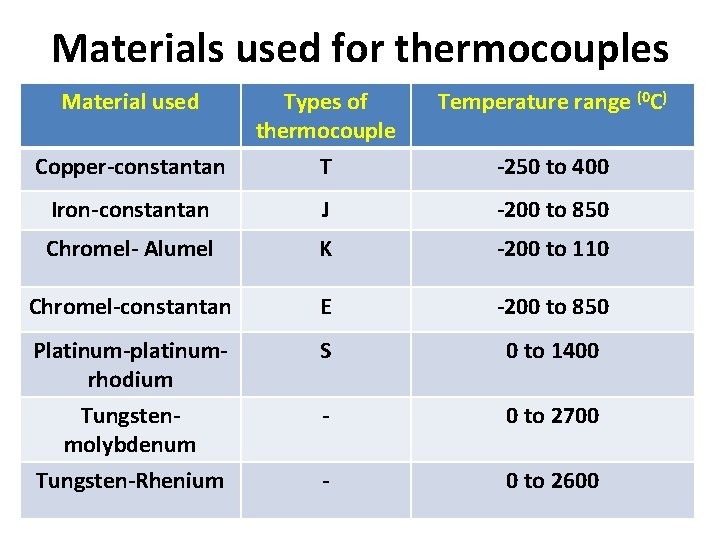
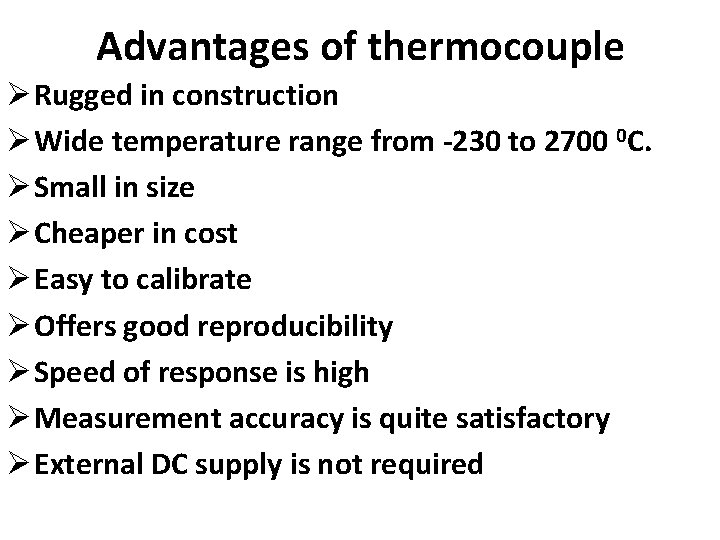
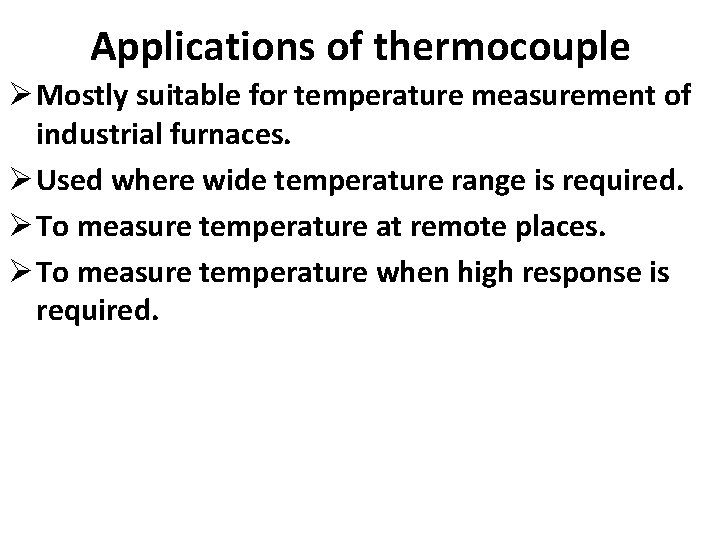
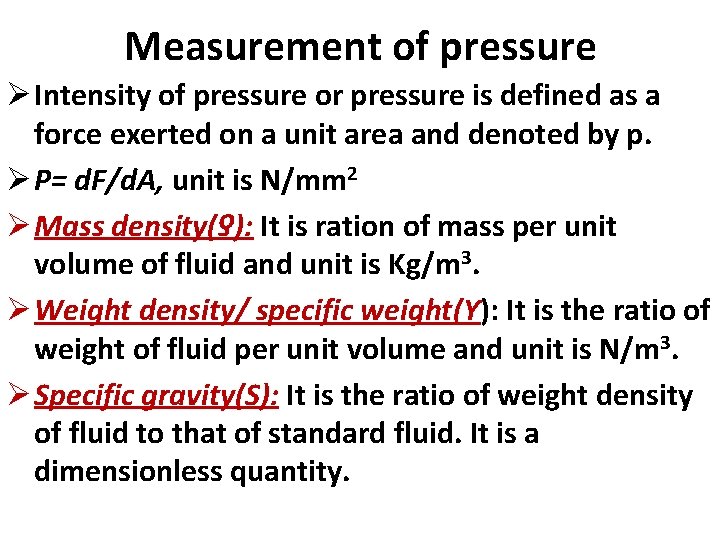
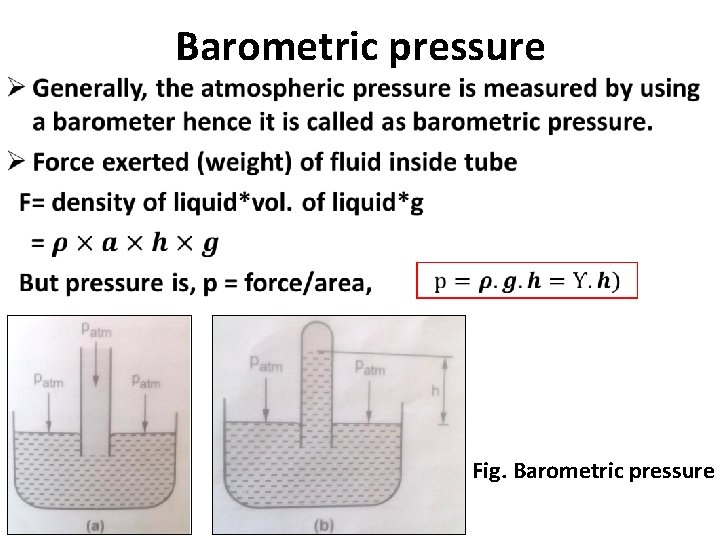
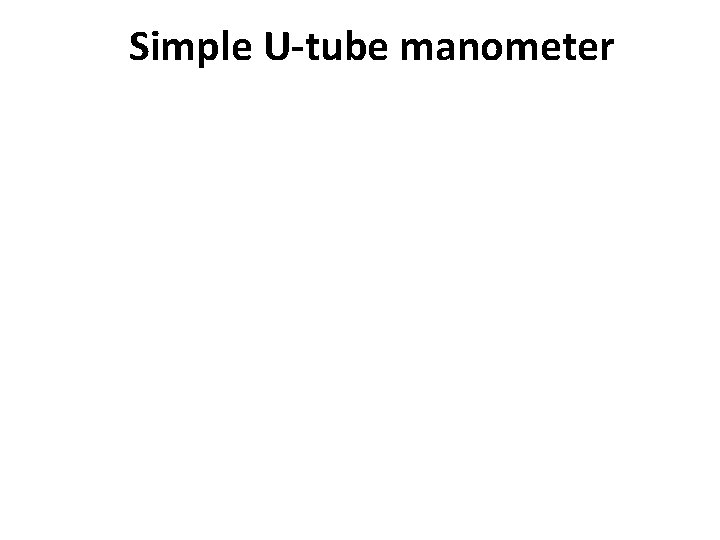
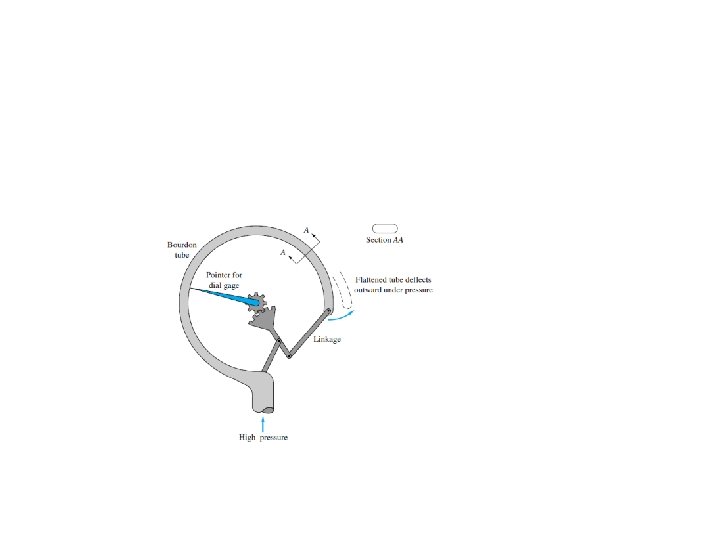
- Slides: 45
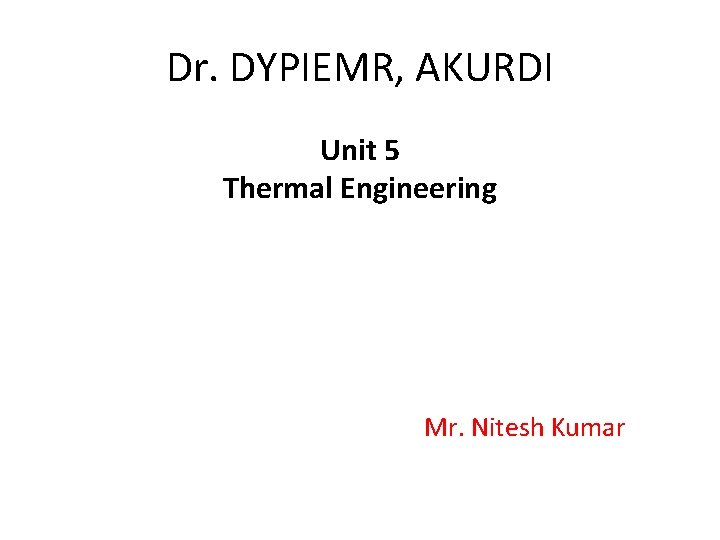
Dr. DYPIEMR, AKURDI Unit 5 Thermal Engineering Mr. Nitesh Kumar
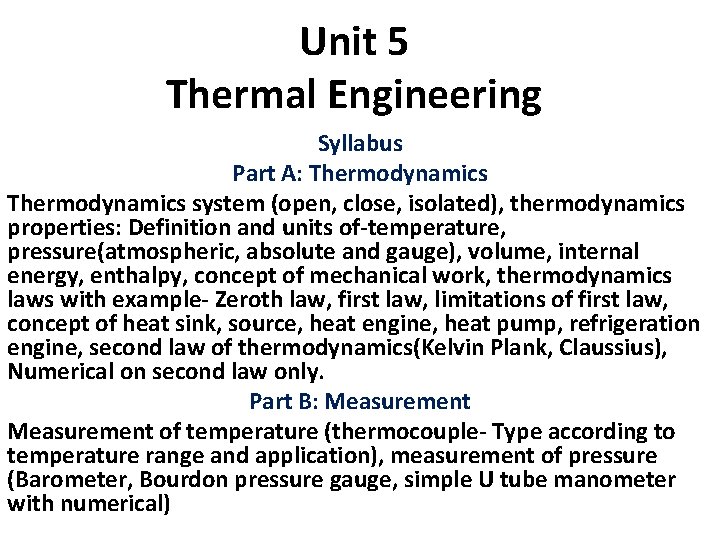
Unit 5 Thermal Engineering Syllabus Part A: Thermodynamics system (open, close, isolated), thermodynamics properties: Definition and units of-temperature, pressure(atmospheric, absolute and gauge), volume, internal energy, enthalpy, concept of mechanical work, thermodynamics laws with example- Zeroth law, first law, limitations of first law, concept of heat sink, source, heat engine, heat pump, refrigeration engine, second law of thermodynamics(Kelvin Plank, Claussius), Numerical on second law only. Part B: Measurement of temperature (thermocouple- Type according to temperature range and application), measurement of pressure (Barometer, Bourdon pressure gauge, simple U tube manometer with numerical)
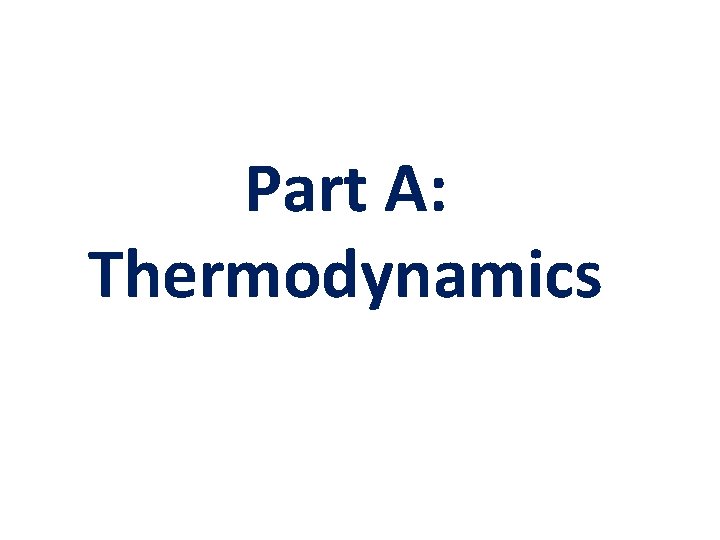
Part A: Thermodynamics
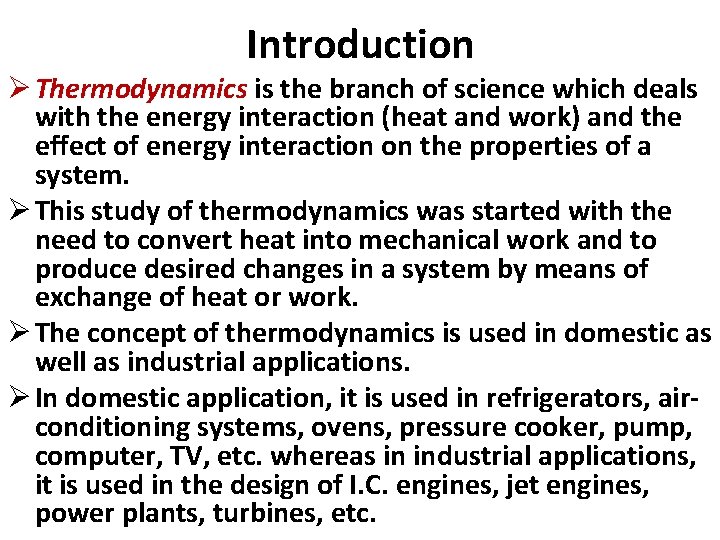
Introduction Ø Thermodynamics is the branch of science which deals with the energy interaction (heat and work) and the effect of energy interaction on the properties of a system. Ø This study of thermodynamics was started with the need to convert heat into mechanical work and to produce desired changes in a system by means of exchange of heat or work. Ø The concept of thermodynamics is used in domestic as well as industrial applications. Ø In domestic application, it is used in refrigerators, airconditioning systems, ovens, pressure cooker, pump, computer, TV, etc. whereas in industrial applications, it is used in the design of I. C. engines, jet engines, power plants, turbines, etc.
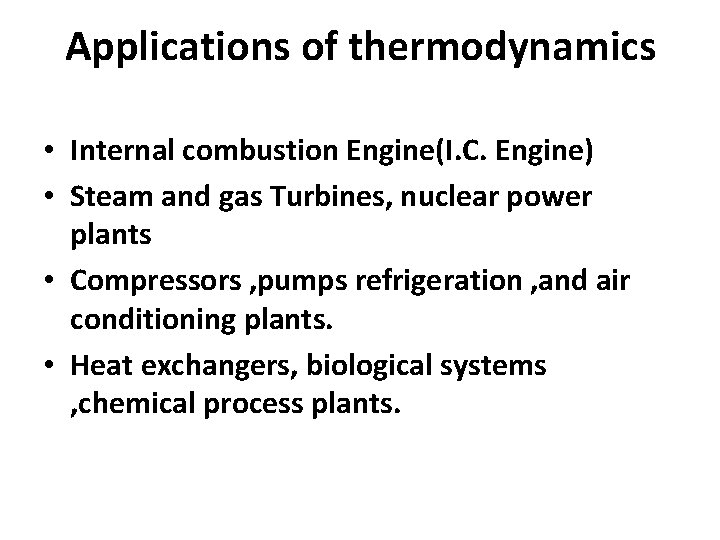
Applications of thermodynamics • Internal combustion Engine(I. C. Engine) • Steam and gas Turbines, nuclear power plants • Compressors , pumps refrigeration , and air conditioning plants. • Heat exchangers, biological systems , chemical process plants.
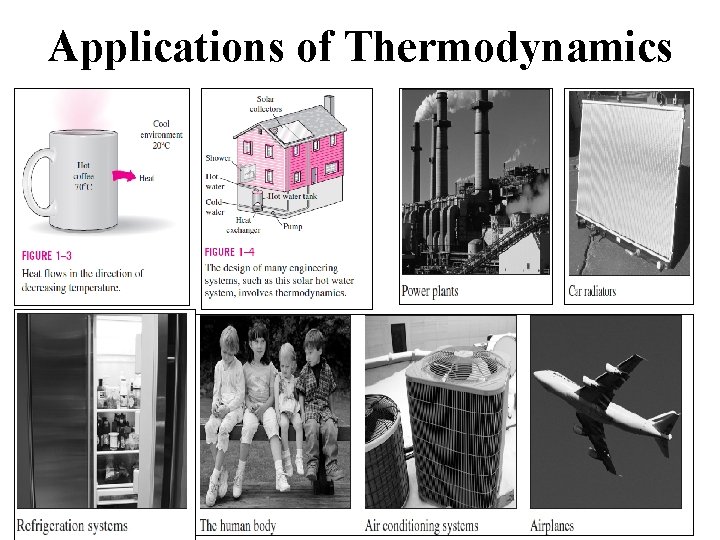
Applications of Thermodynamics
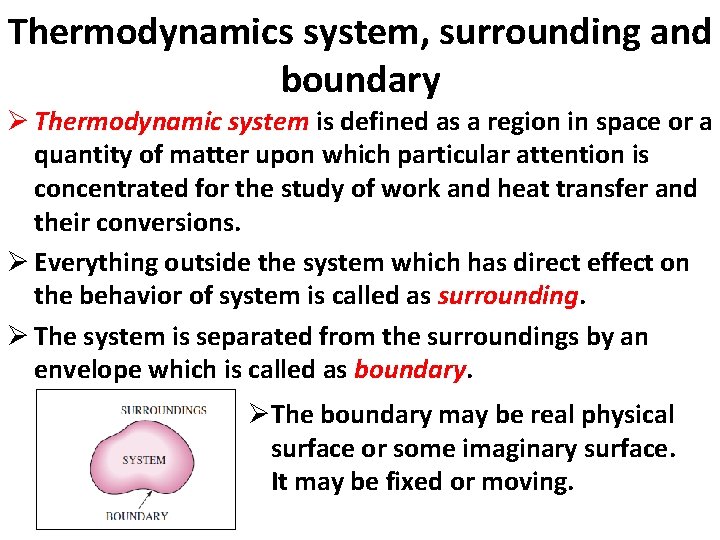
Thermodynamics system, surrounding and boundary Ø Thermodynamic system is defined as a region in space or a quantity of matter upon which particular attention is concentrated for the study of work and heat transfer and their conversions. Ø Everything outside the system which has direct effect on the behavior of system is called as surrounding. Ø The system is separated from the surroundings by an envelope which is called as boundary. ØThe boundary may be real physical surface or some imaginary surface. It may be fixed or moving.
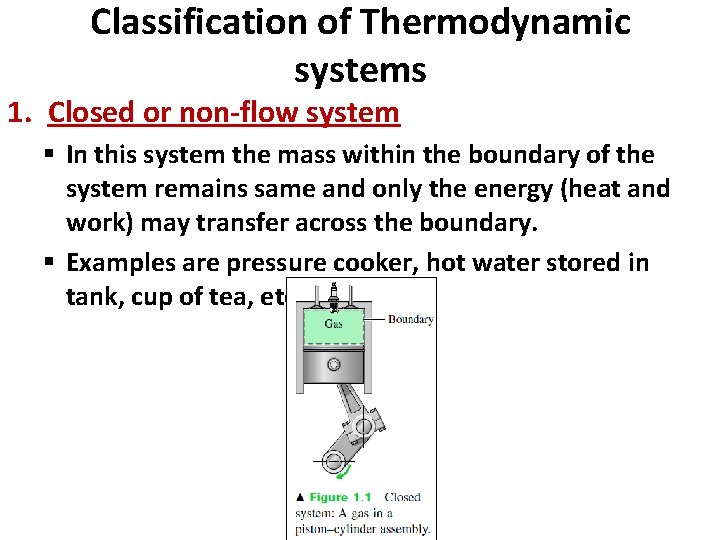
Classification of Thermodynamic systems 1. Closed or non-flow system § In this system the mass within the boundary of the system remains same and only the energy (heat and work) may transfer across the boundary. § Examples are pressure cooker, hot water stored in tank, cup of tea, etc
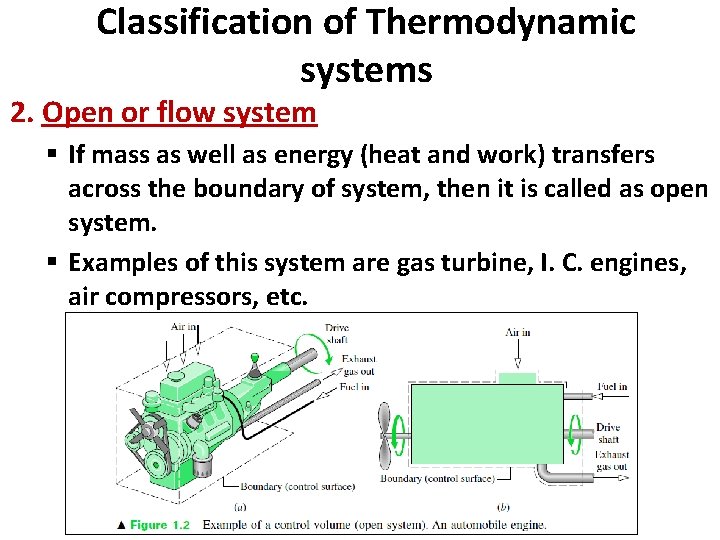
Classification of Thermodynamic systems 2. Open or flow system § If mass as well as energy (heat and work) transfers across the boundary of system, then it is called as open system. § Examples of this system are gas turbine, I. C. engines, air compressors, etc.
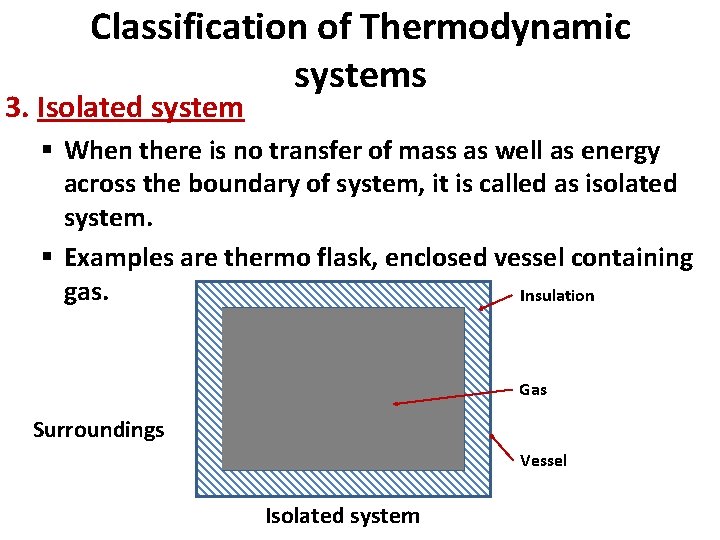
Classification of Thermodynamic systems 3. Isolated system § When there is no transfer of mass as well as energy across the boundary of system, it is called as isolated system. § Examples are thermo flask, enclosed vessel containing gas. Insulation Gas Surroundings Vessel Isolated system
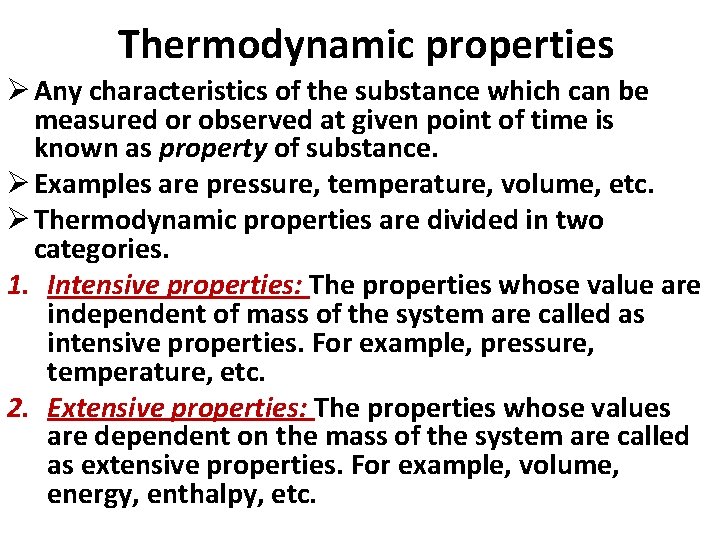
Thermodynamic properties Ø Any characteristics of the substance which can be measured or observed at given point of time is known as property of substance. Ø Examples are pressure, temperature, volume, etc. Ø Thermodynamic properties are divided in two categories. 1. Intensive properties: The properties whose value are independent of mass of the system are called as intensive properties. For example, pressure, temperature, etc. 2. Extensive properties: The properties whose values are dependent on the mass of the system are called as extensive properties. For example, volume, energy, enthalpy, etc.
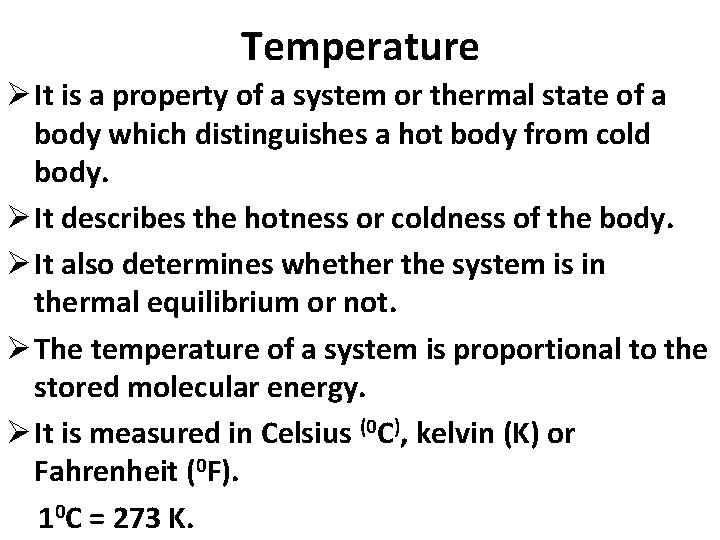
Temperature Ø It is a property of a system or thermal state of a body which distinguishes a hot body from cold body. Ø It describes the hotness or coldness of the body. Ø It also determines whether the system is in thermal equilibrium or not. Ø The temperature of a system is proportional to the stored molecular energy. Ø It is measured in Celsius (0 C), kelvin (K) or Fahrenheit (0 F). 10 C = 273 K.
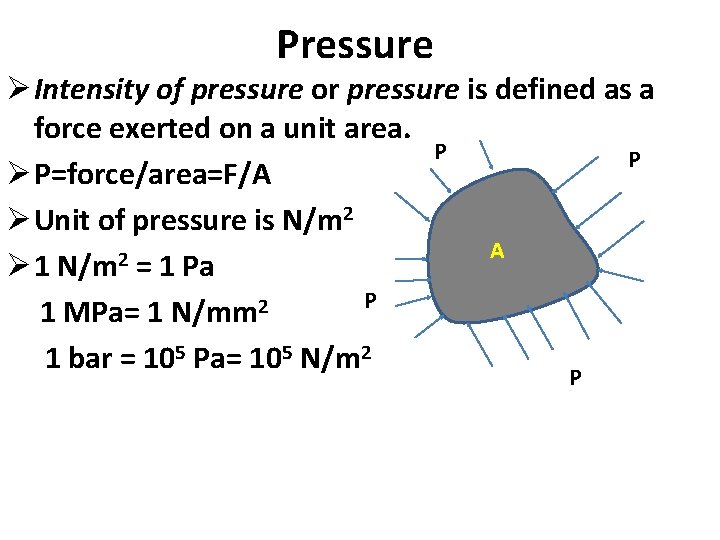
Pressure Ø Intensity of pressure or pressure is defined as a force exerted on a unit area. P P Ø P=force/area=F/A Ø Unit of pressure is N/m 2 A 2 Ø 1 N/m = 1 Pa P 2 1 MPa= 1 N/mm 1 bar = 105 Pa= 105 N/m 2 P
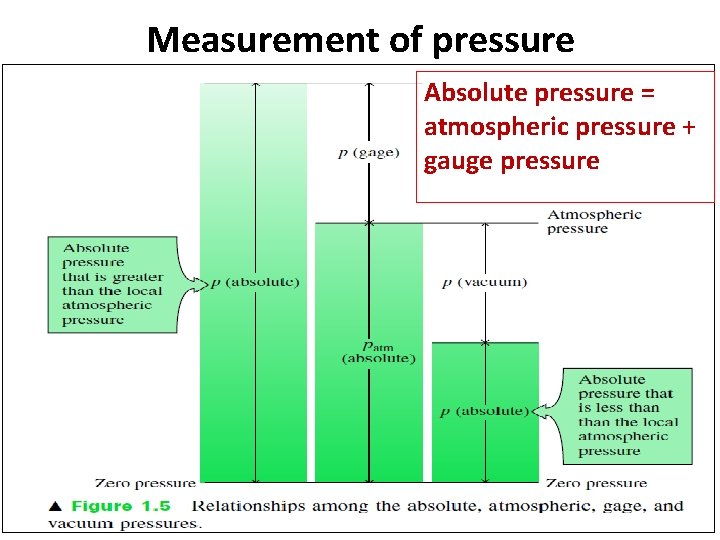
Measurement of pressure Absolute pressure = atmospheric pressure + gauge pressure
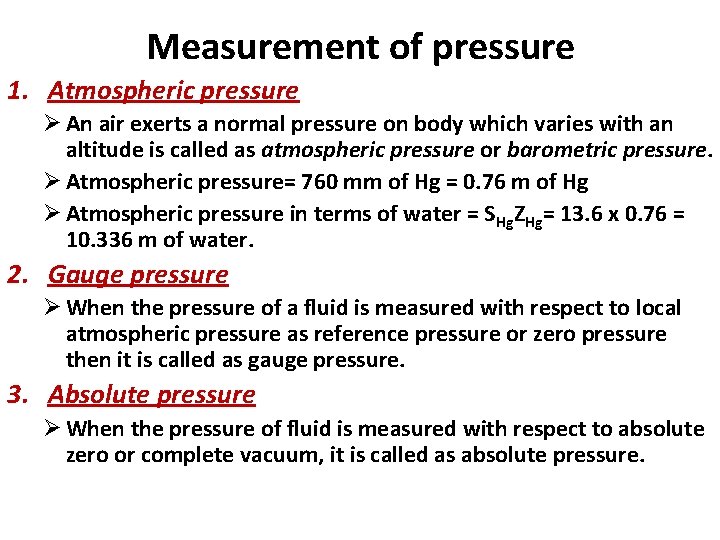
Measurement of pressure 1. Atmospheric pressure Ø An air exerts a normal pressure on body which varies with an altitude is called as atmospheric pressure or barometric pressure. Ø Atmospheric pressure= 760 mm of Hg = 0. 76 m of Hg Ø Atmospheric pressure in terms of water = SHg. ZHg= 13. 6 x 0. 76 = 10. 336 m of water. 2. Gauge pressure Ø When the pressure of a fluid is measured with respect to local atmospheric pressure as reference pressure or zero pressure then it is called as gauge pressure. 3. Absolute pressure Ø When the pressure of fluid is measured with respect to absolute zero or complete vacuum, it is called as absolute pressure.
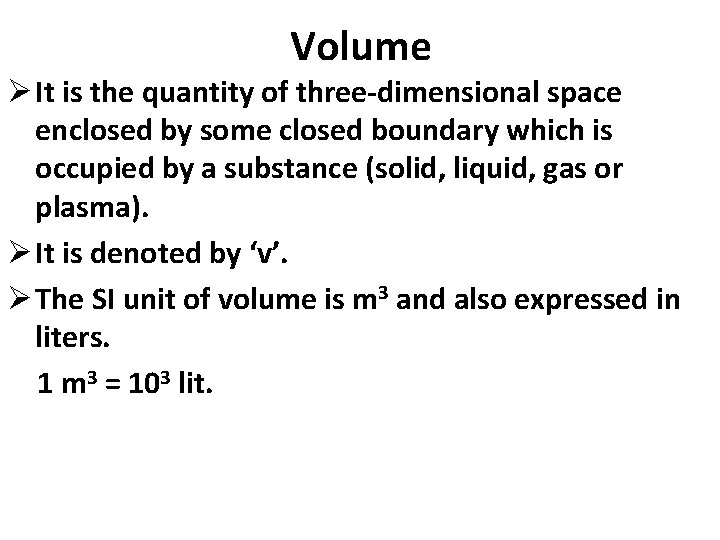
Volume Ø It is the quantity of three-dimensional space enclosed by some closed boundary which is occupied by a substance (solid, liquid, gas or plasma). Ø It is denoted by ‘v’. Ø The SI unit of volume is m 3 and also expressed in liters. 1 m 3 = 103 lit.
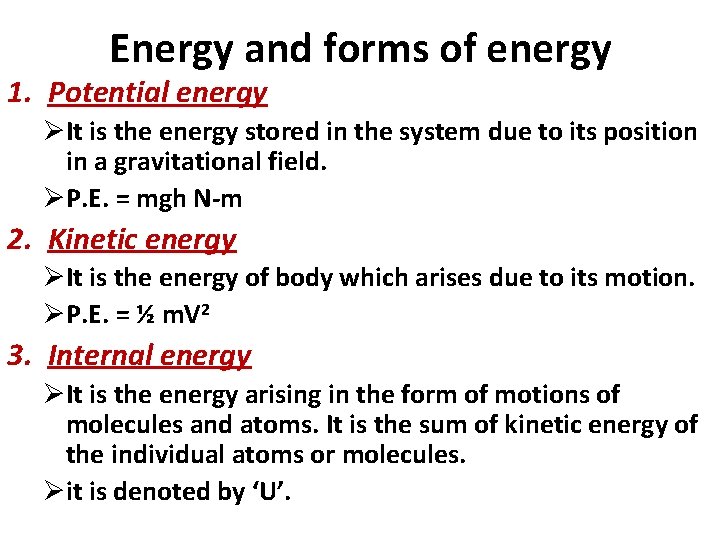
Energy and forms of energy 1. Potential energy ØIt is the energy stored in the system due to its position in a gravitational field. ØP. E. = mgh N-m 2. Kinetic energy ØIt is the energy of body which arises due to its motion. ØP. E. = ½ m. V 2 3. Internal energy ØIt is the energy arising in the form of motions of molecules and atoms. It is the sum of kinetic energy of the individual atoms or molecules. Øit is denoted by ‘U’.
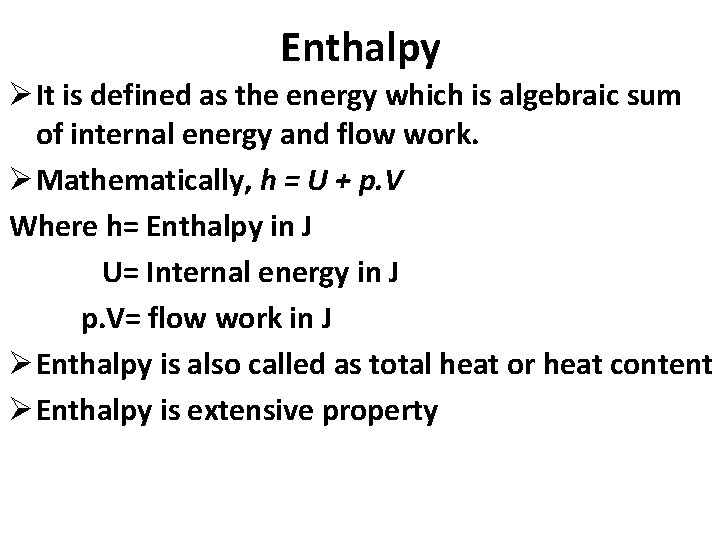
Enthalpy Ø It is defined as the energy which is algebraic sum of internal energy and flow work. Ø Mathematically, h = U + p. V Where h= Enthalpy in J U= Internal energy in J p. V= flow work in J Ø Enthalpy is also called as total heat or heat content Ø Enthalpy is extensive property
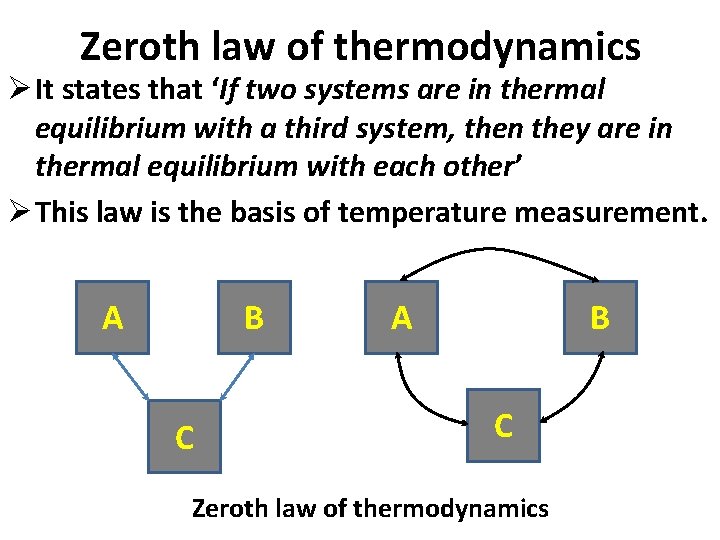
Zeroth law of thermodynamics Ø It states that ‘If two systems are in thermal equilibrium with a third system, then they are in thermal equilibrium with each other’ Ø This law is the basis of temperature measurement. A B C Zeroth law of thermodynamics
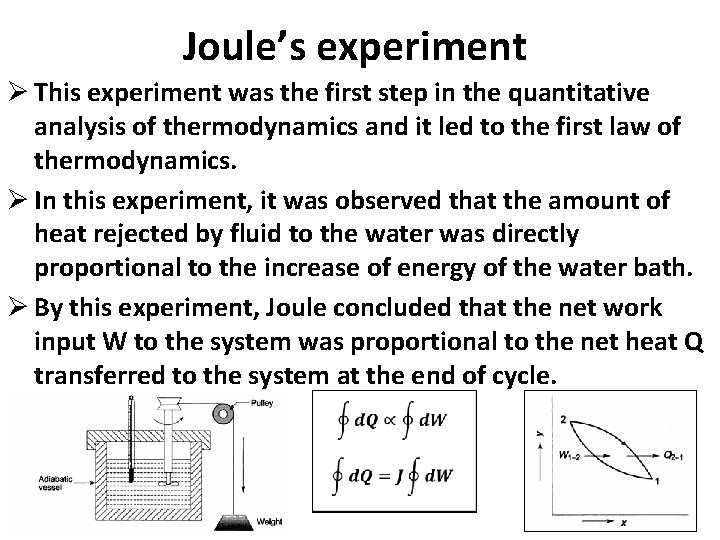
Joule’s experiment Ø This experiment was the first step in the quantitative analysis of thermodynamics and it led to the first law of thermodynamics. Ø In this experiment, it was observed that the amount of heat rejected by fluid to the water was directly proportional to the increase of energy of the water bath. Ø By this experiment, Joule concluded that the net work input W to the system was proportional to the net heat Q transferred to the system at the end of cycle.
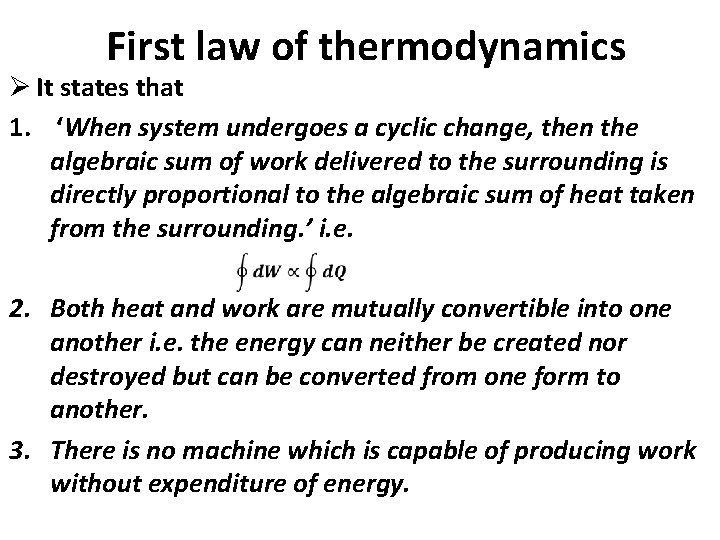
First law of thermodynamics Ø It states that 1. ‘When system undergoes a cyclic change, then the algebraic sum of work delivered to the surrounding is directly proportional to the algebraic sum of heat taken from the surrounding. ’ i. e. 2. Both heat and work are mutually convertible into one another i. e. the energy can neither be created nor destroyed but can be converted from one form to another. 3. There is no machine which is capable of producing work without expenditure of energy.
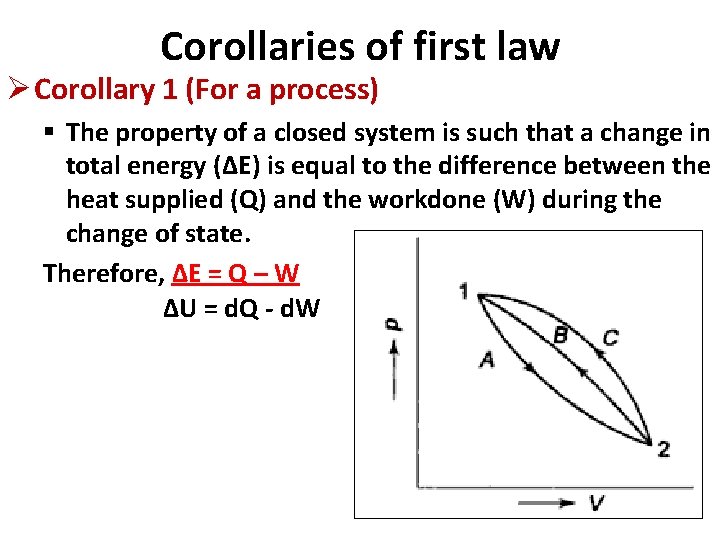
Corollaries of first law Ø Corollary 1 (For a process) § The property of a closed system is such that a change in total energy (ΔE) is equal to the difference between the heat supplied (Q) and the workdone (W) during the change of state. Therefore, ΔE = Q – W ΔU = d. Q - d. W
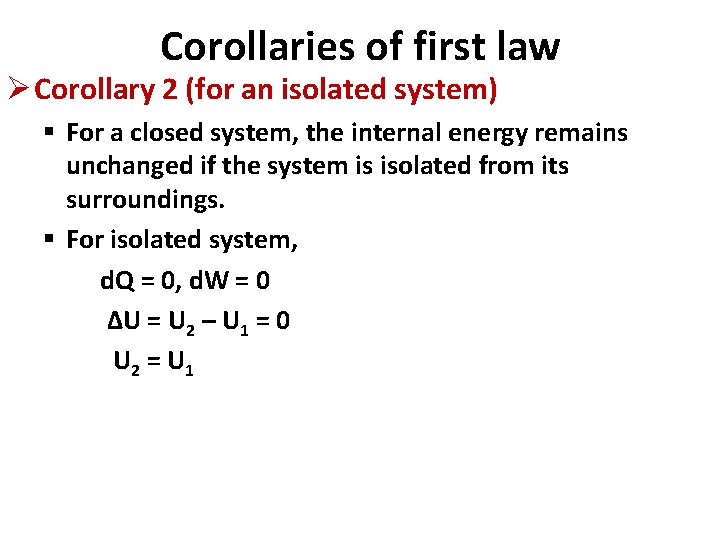
Corollaries of first law Ø Corollary 2 (for an isolated system) § For a closed system, the internal energy remains unchanged if the system is isolated from its surroundings. § For isolated system, d. Q = 0, d. W = 0 ΔU = U 2 – U 1 = 0 U 2 = U 1
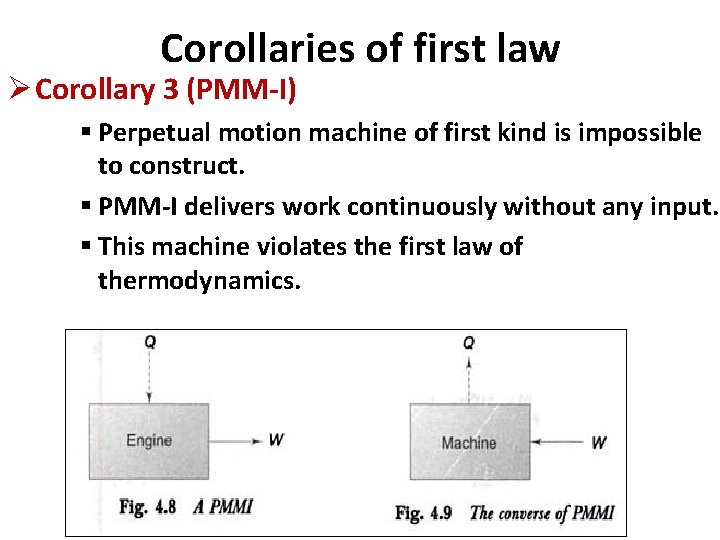
Corollaries of first law Ø Corollary 3 (PMM-I) § Perpetual motion machine of first kind is impossible to construct. § PMM-I delivers work continuously without any input. § This machine violates the first law of thermodynamics.
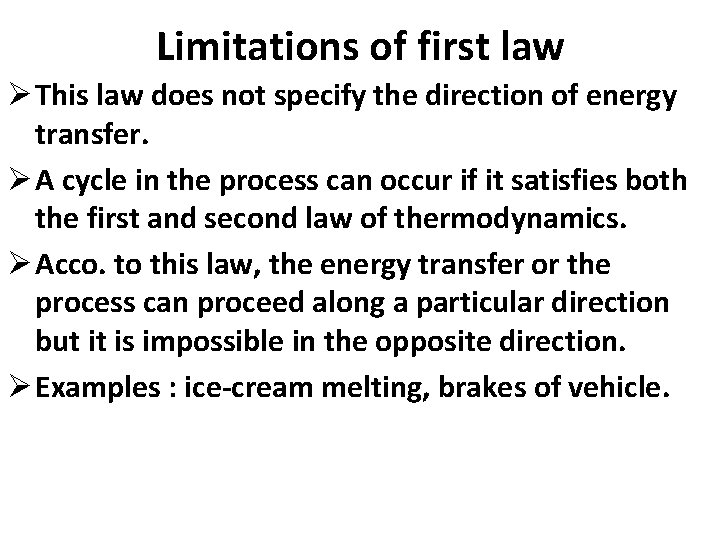
Limitations of first law Ø This law does not specify the direction of energy transfer. Ø A cycle in the process can occur if it satisfies both the first and second law of thermodynamics. Ø Acco. to this law, the energy transfer or the process can proceed along a particular direction but it is impossible in the opposite direction. Ø Examples : ice-cream melting, brakes of vehicle.
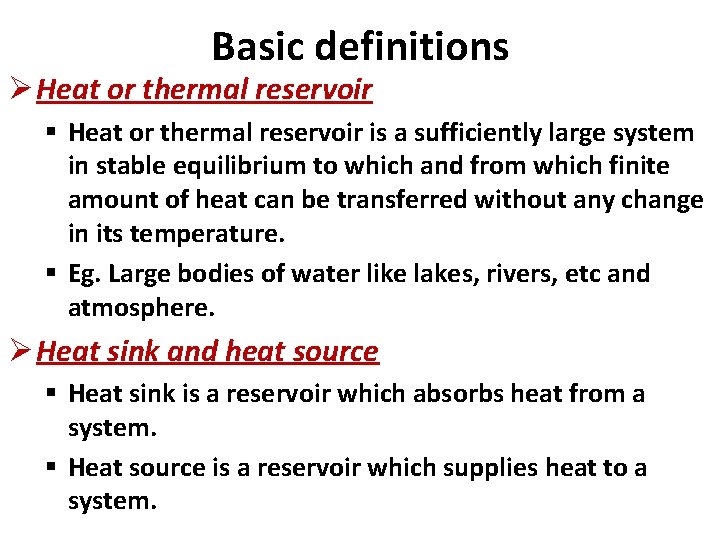
Basic definitions Ø Heat or thermal reservoir § Heat or thermal reservoir is a sufficiently large system in stable equilibrium to which and from which finite amount of heat can be transferred without any change in its temperature. § Eg. Large bodies of water like lakes, rivers, etc and atmosphere. Ø Heat sink and heat source § Heat sink is a reservoir which absorbs heat from a system. § Heat source is a reservoir which supplies heat to a system.
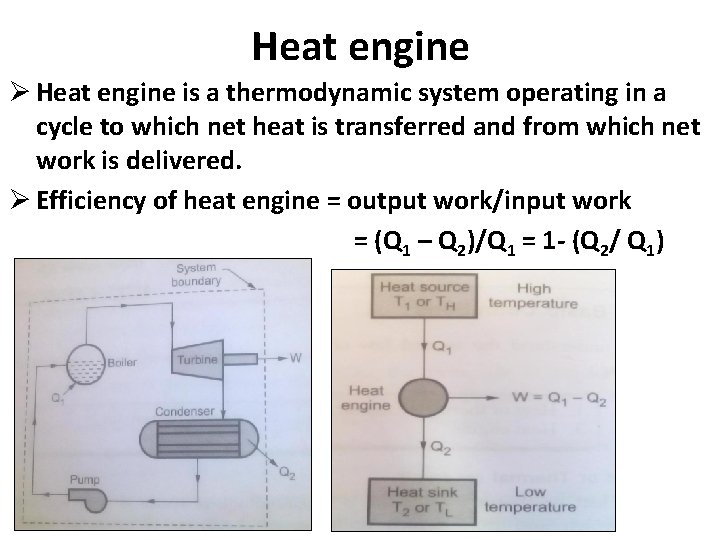
Heat engine Ø Heat engine is a thermodynamic system operating in a cycle to which net heat is transferred and from which net work is delivered. Ø Efficiency of heat engine = output work/input work = (Q 1 – Q 2)/Q 1 = 1 - (Q 2/ Q 1)
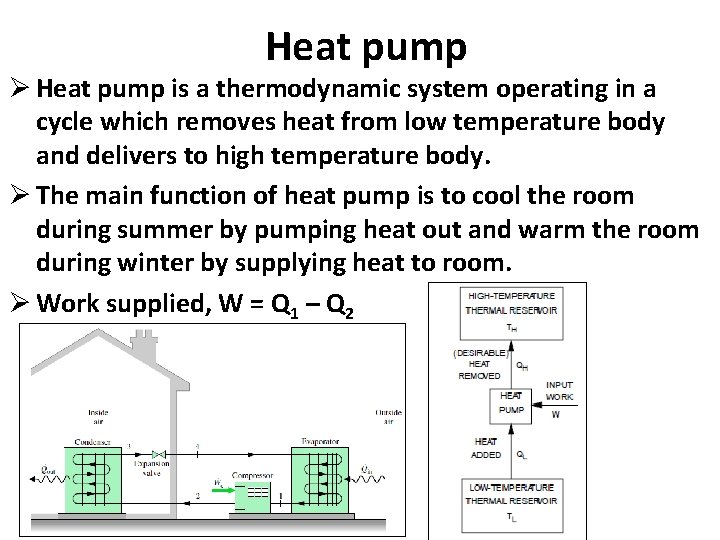
Heat pump Ø Heat pump is a thermodynamic system operating in a cycle which removes heat from low temperature body and delivers to high temperature body. Ø The main function of heat pump is to cool the room during summer by pumping heat out and warm the room during winter by supplying heat to room. Ø Work supplied, W = Q 1 – Q 2
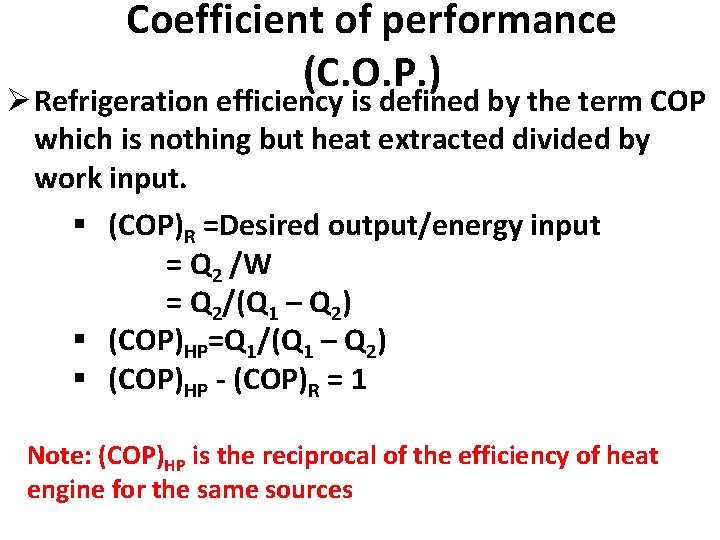
Coefficient of performance (C. O. P. ) Ø Refrigeration efficiency is defined by the term COP which is nothing but heat extracted divided by work input. § (COP)R =Desired output/energy input = Q 2 /W = Q 2/(Q 1 – Q 2) § (COP)HP=Q 1/(Q 1 – Q 2) § (COP)HP - (COP)R = 1 Note: (COP)HP is the reciprocal of the efficiency of heat engine for the same sources
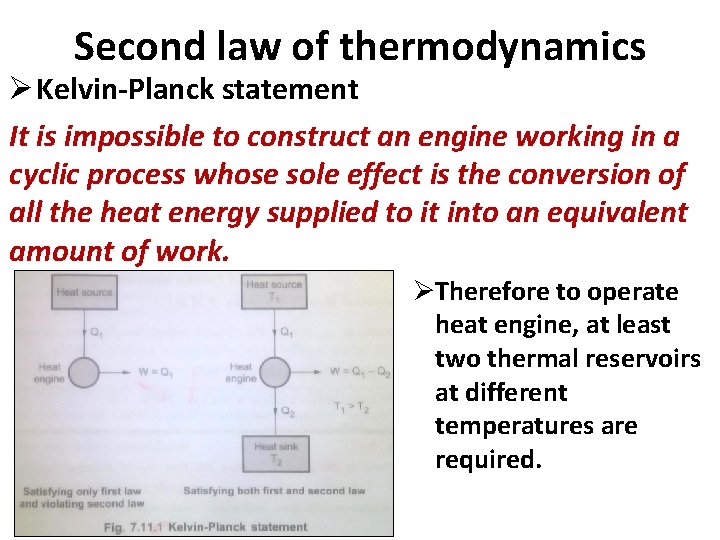
Second law of thermodynamics Ø Kelvin-Planck statement It is impossible to construct an engine working in a cyclic process whose sole effect is the conversion of all the heat energy supplied to it into an equivalent amount of work. ØTherefore to operate heat engine, at least two thermal reservoirs at different temperatures are required.
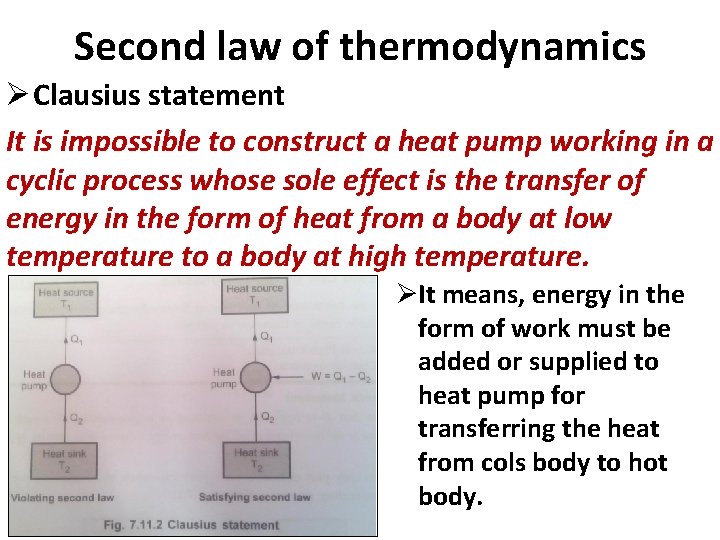
Second law of thermodynamics Ø Clausius statement It is impossible to construct a heat pump working in a cyclic process whose sole effect is the transfer of energy in the form of heat from a body at low temperature to a body at high temperature. ØIt means, energy in the form of work must be added or supplied to heat pump for transferring the heat from cols body to hot body.
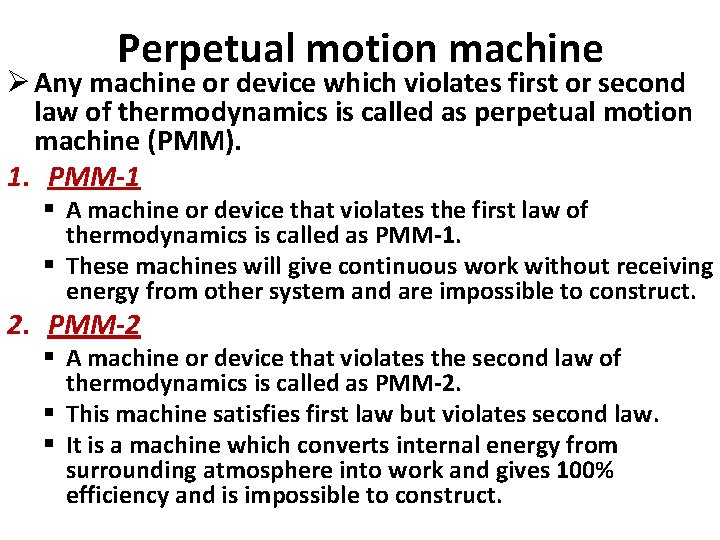
Perpetual motion machine Ø Any machine or device which violates first or second law of thermodynamics is called as perpetual motion machine (PMM). 1. PMM-1 § A machine or device that violates the first law of thermodynamics is called as PMM-1. § These machines will give continuous work without receiving energy from other system and are impossible to construct. 2. PMM-2 § A machine or device that violates the second law of thermodynamics is called as PMM-2. § This machine satisfies first law but violates second law. § It is a machine which converts internal energy from surrounding atmosphere into work and gives 100% efficiency and is impossible to construct.
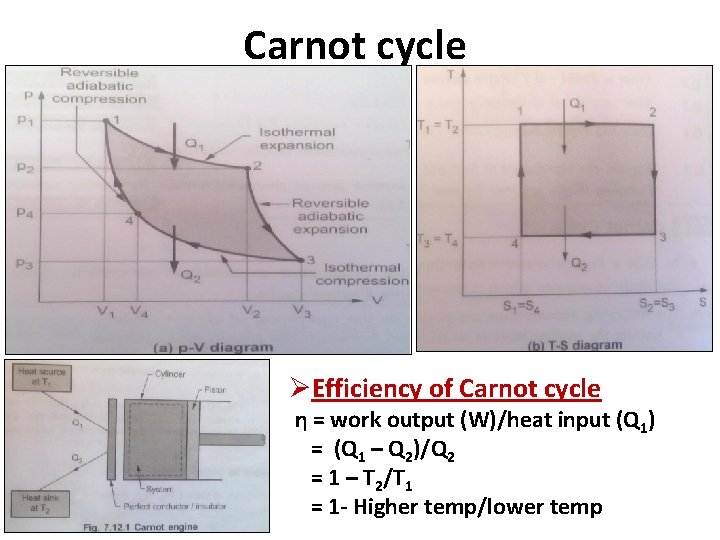
Carnot cycle ØEfficiency of Carnot cycle η = work output (W)/heat input (Q 1) = (Q 1 – Q 2)/Q 2 = 1 – T 2/T 1 = 1 - Higher temp/lower temp
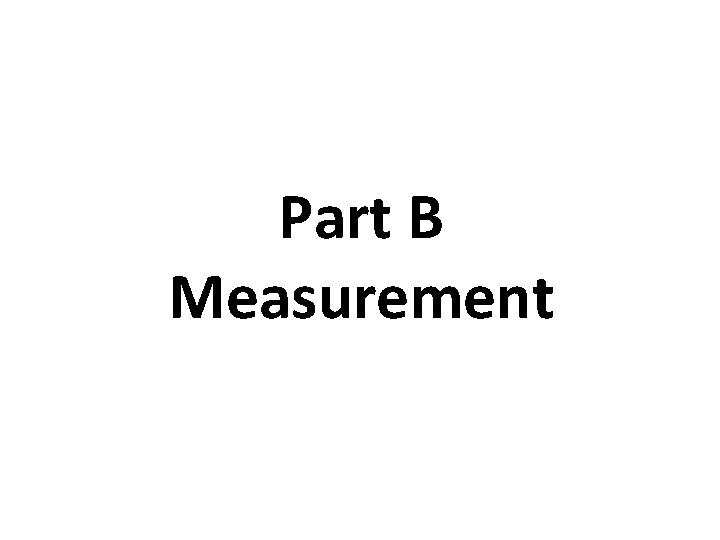
Part B Measurement
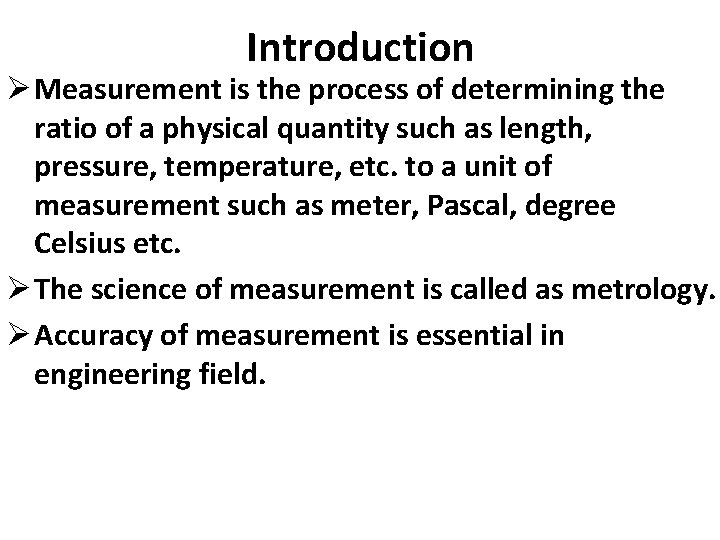
Introduction Ø Measurement is the process of determining the ratio of a physical quantity such as length, pressure, temperature, etc. to a unit of measurement such as meter, Pascal, degree Celsius etc. Ø The science of measurement is called as metrology. Ø Accuracy of measurement is essential in engineering field.
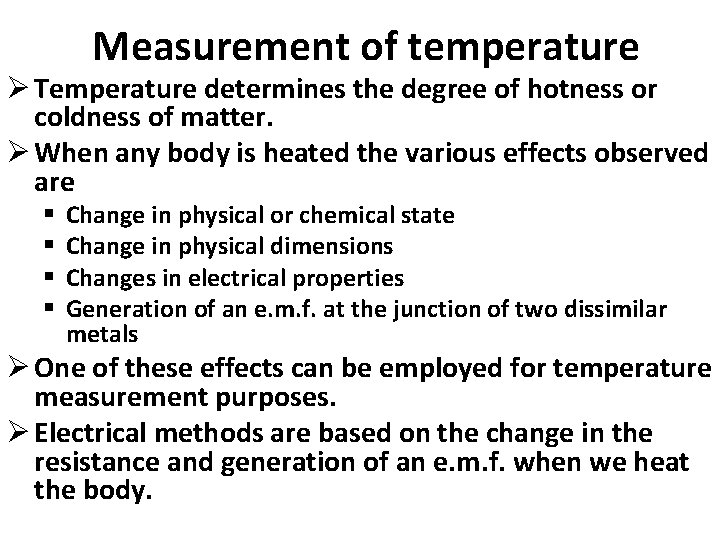
Measurement of temperature Ø Temperature determines the degree of hotness or coldness of matter. Ø When any body is heated the various effects observed are § § Change in physical or chemical state Change in physical dimensions Changes in electrical properties Generation of an e. m. f. at the junction of two dissimilar metals Ø One of these effects can be employed for temperature measurement purposes. Ø Electrical methods are based on the change in the resistance and generation of an e. m. f. when we heat the body.
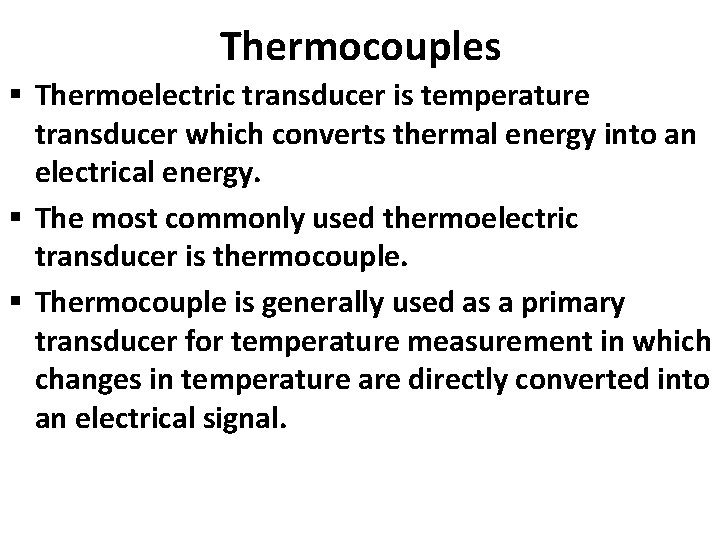
Thermocouples § Thermoelectric transducer is temperature transducer which converts thermal energy into an electrical energy. § The most commonly used thermoelectric transducer is thermocouple. § Thermocouple is generally used as a primary transducer for temperature measurement in which changes in temperature are directly converted into an electrical signal.
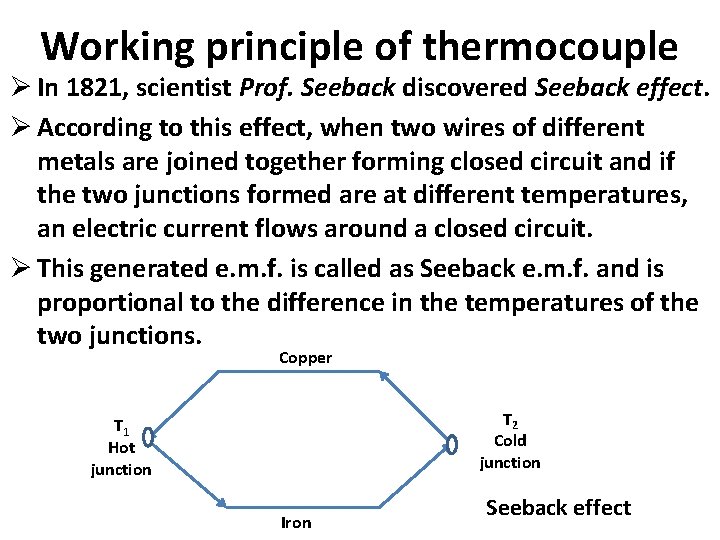
Working principle of thermocouple Ø In 1821, scientist Prof. Seeback discovered Seeback effect. Ø According to this effect, when two wires of different metals are joined together forming closed circuit and if the two junctions formed are at different temperatures, an electric current flows around a closed circuit. Ø This generated e. m. f. is called as Seeback e. m. f. and is proportional to the difference in the temperatures of the two junctions. Copper T 2 Cold junction T 1 Hot junction Iron Seeback effect
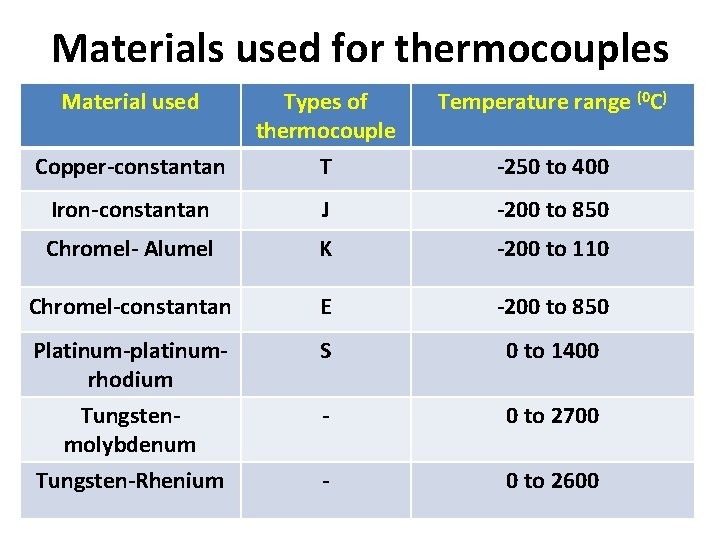
Materials used for thermocouples Material used Temperature range (0 C) Copper-constantan Types of thermocouple T Iron-constantan J -200 to 850 Chromel- Alumel K -200 to 110 Chromel-constantan E -200 to 850 Platinum-platinumrhodium Tungstenmolybdenum S 0 to 1400 - 0 to 2700 Tungsten-Rhenium - 0 to 2600 -250 to 400
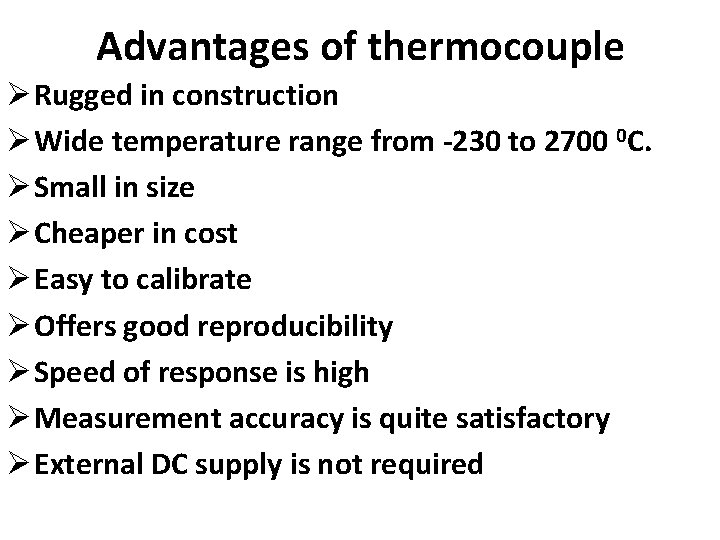
Advantages of thermocouple Ø Rugged in construction Ø Wide temperature range from -230 to 2700 0 C. Ø Small in size Ø Cheaper in cost Ø Easy to calibrate Ø Offers good reproducibility Ø Speed of response is high Ø Measurement accuracy is quite satisfactory Ø External DC supply is not required
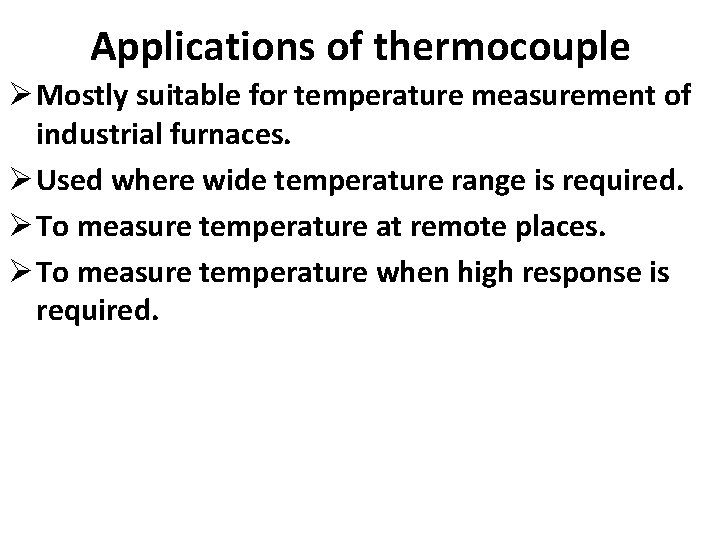
Applications of thermocouple Ø Mostly suitable for temperature measurement of industrial furnaces. Ø Used where wide temperature range is required. Ø To measure temperature at remote places. Ø To measure temperature when high response is required.
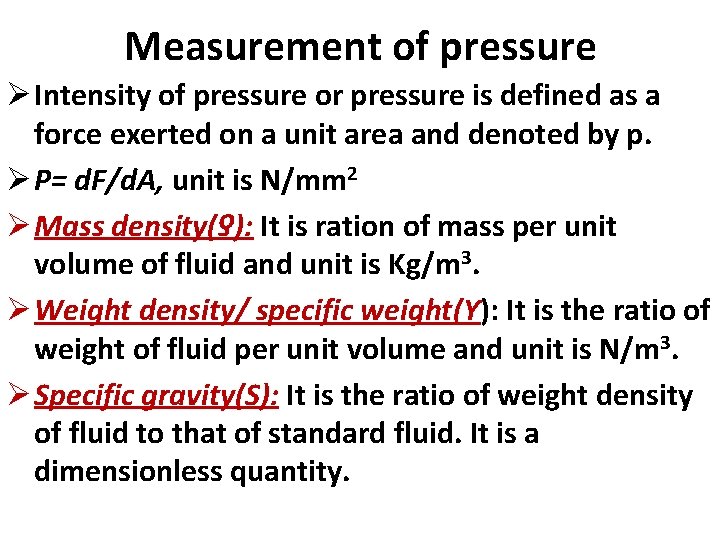
Measurement of pressure Ø Intensity of pressure or pressure is defined as a force exerted on a unit area and denoted by p. Ø P= d. F/d. A, unit is N/mm 2 Ø Mass density(ƍ): It is ration of mass per unit volume of fluid and unit is Kg/m 3. Ø Weight density/ specific weight(Ƴ): It is the ratio of weight of fluid per unit volume and unit is N/m 3. Ø Specific gravity(S): It is the ratio of weight density of fluid to that of standard fluid. It is a dimensionless quantity.
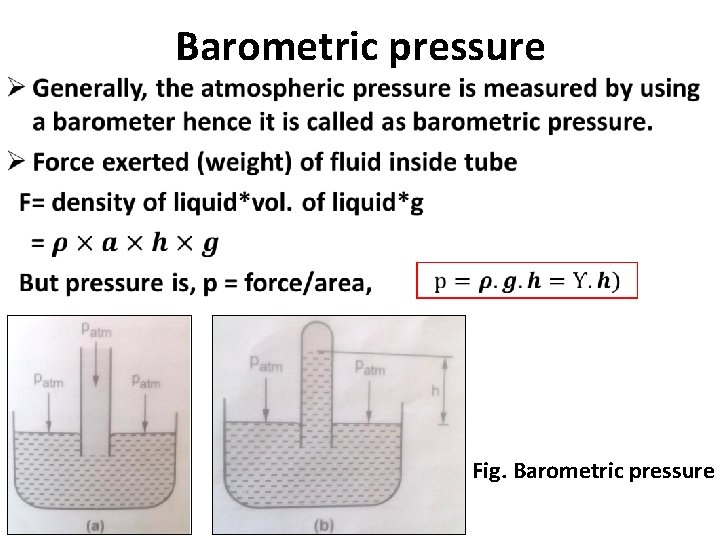
• Barometric pressure Fig. Barometric pressure
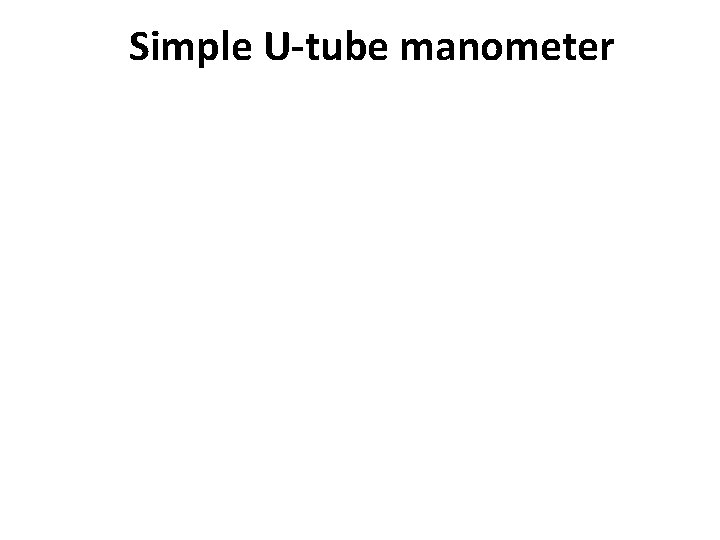
Simple U-tube manometer
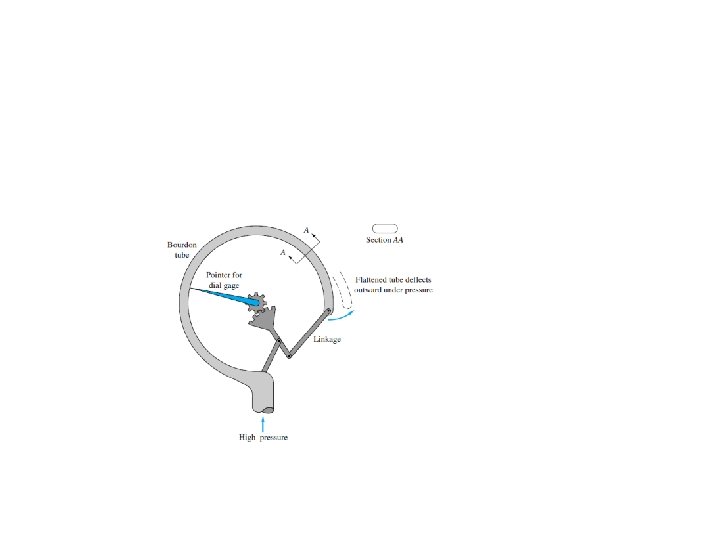
Types of belt drive
Section 3 using heat worksheet answers
Thermal transfer vs direct thermal printing
Thermal engineering basics
Hy time
Unit 6 review questions
Low heat capacity
Thermal expansion in liquids
Si unit of specific heat
Dimensions of thermal conductivity
System procurement process in software engineering
Forward engineering in software engineering
Principles of complex systems for systems engineering
Elegant systems
Forward and reverse engineering
Unit 4 engineering design
Btec sport level 3 unit 2
Btec level 3 engineering unit 1 past papers
Unit 2 communications for engineering technicians
Metode pembiayaan semi langsung
Unit 4 lesson 7 right triangles and trigonometry unit test
Si unit to english unit
Unit 1 test algebra 2 answers
Contoh soal perhitungan unit cost rumah sakit
Unit process and unit operation
Difference between unit process and unit operation
Kerangka konseptual standar akuntansi pemerintahan
Steady state thermal analysis
Thermal inertia definition
Thermal expansion examples
Thermal stability of transistor
What is the difference between thermal energy and heat?
How is thermal energy transferred?
Thermal lift
Warm chain
Specific heat capacity graph
Difference between heat and thermal energy
Difference between heat and thermal energy
Thermal mass flow meter calibration
Thermal press hair
Thermal expansion examples
What is heat energy?
Thermal energy depends on
What is todays temperature
Define thermal energy
Difference between heat and thermal energy