Colloidal semiconductor quantum dots QDs for advanced optical
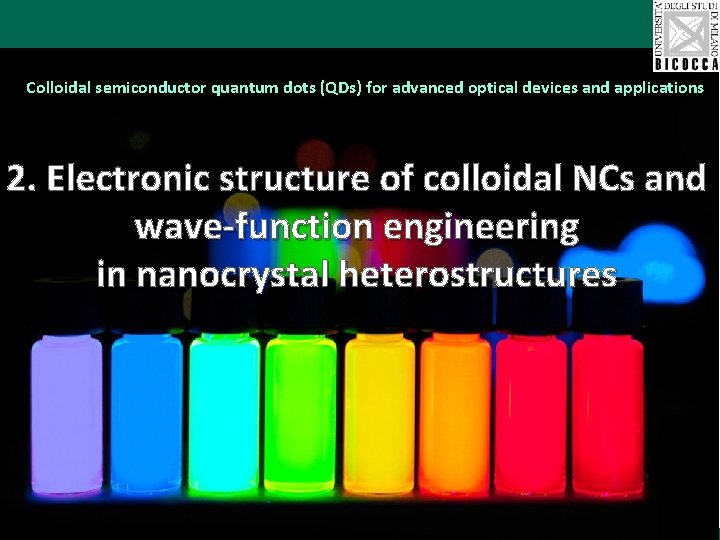
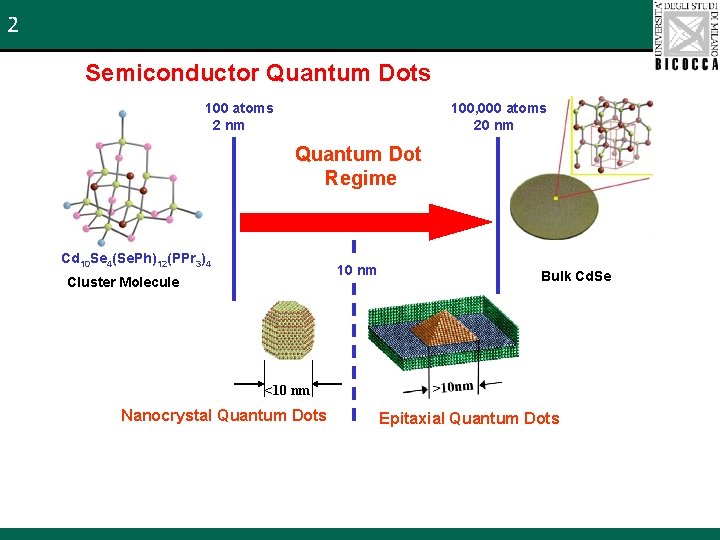
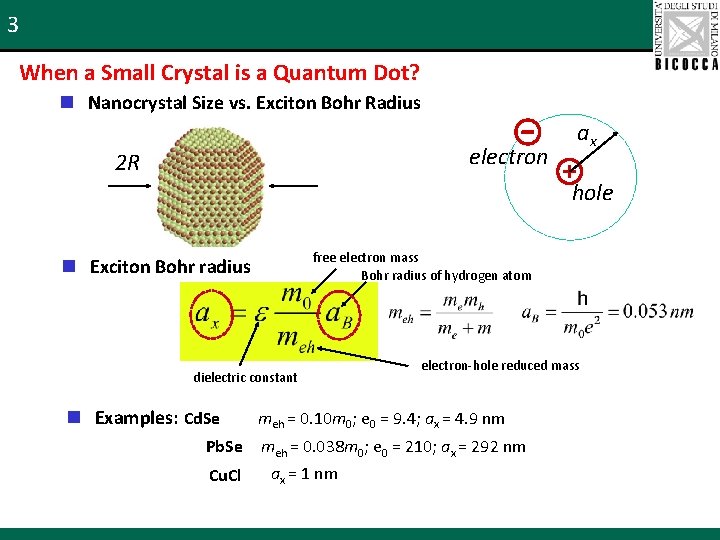
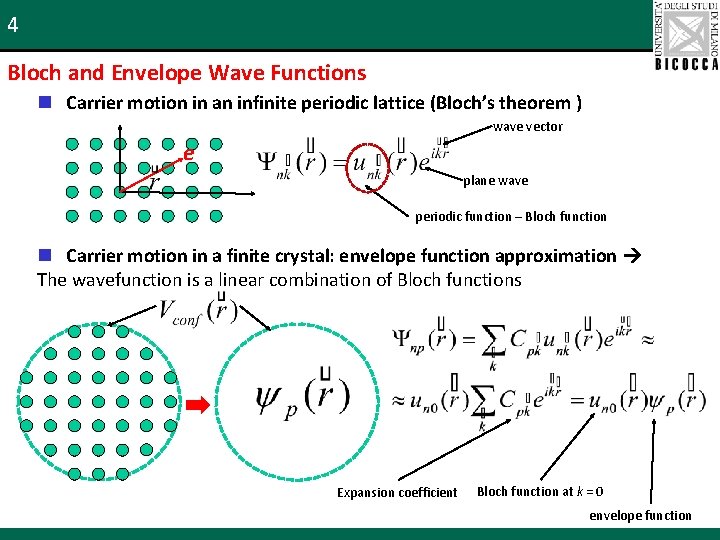
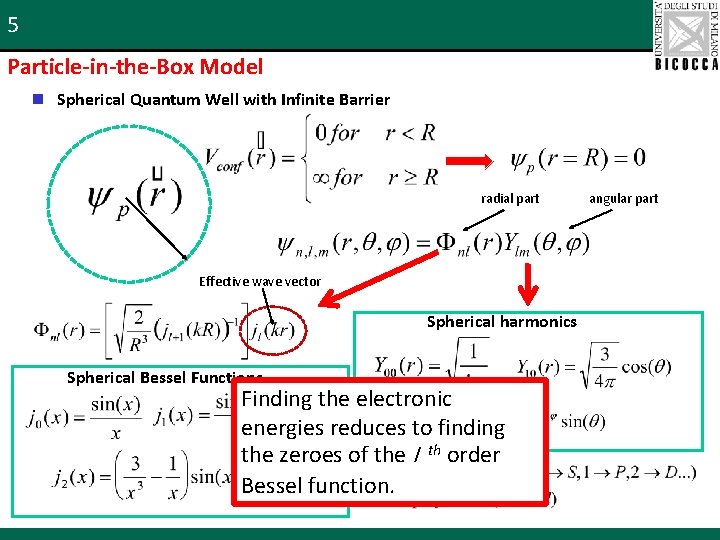
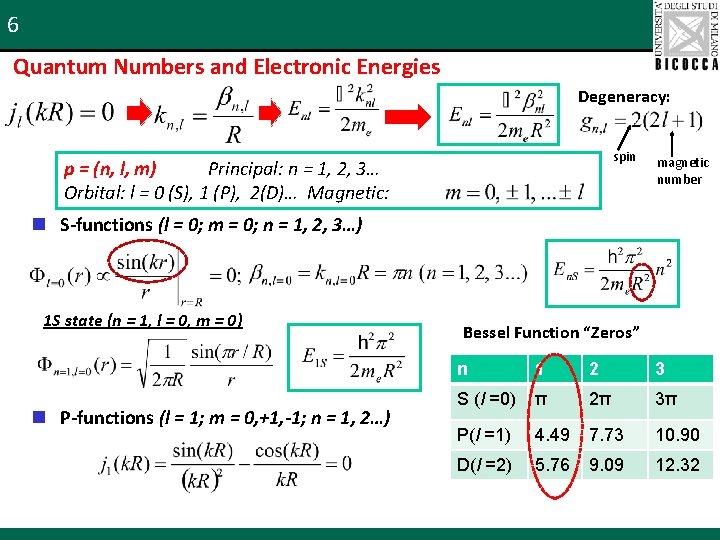
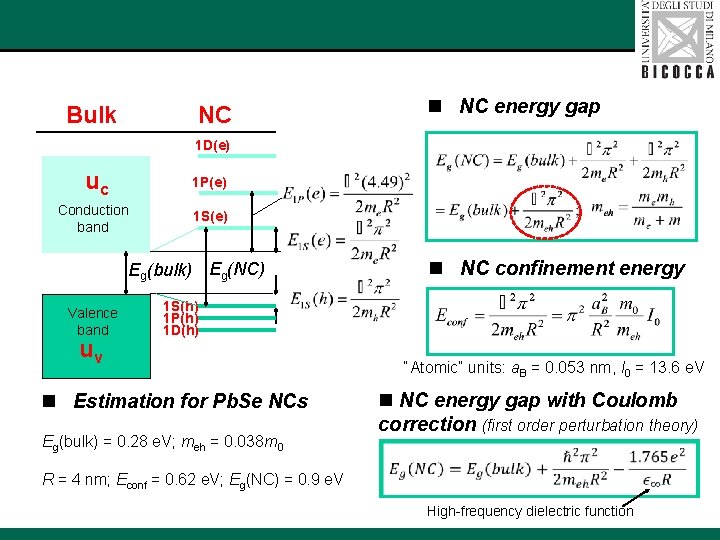
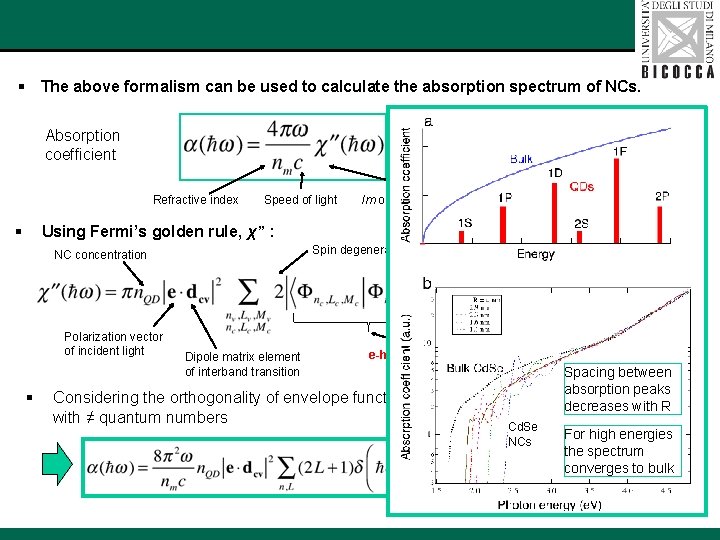
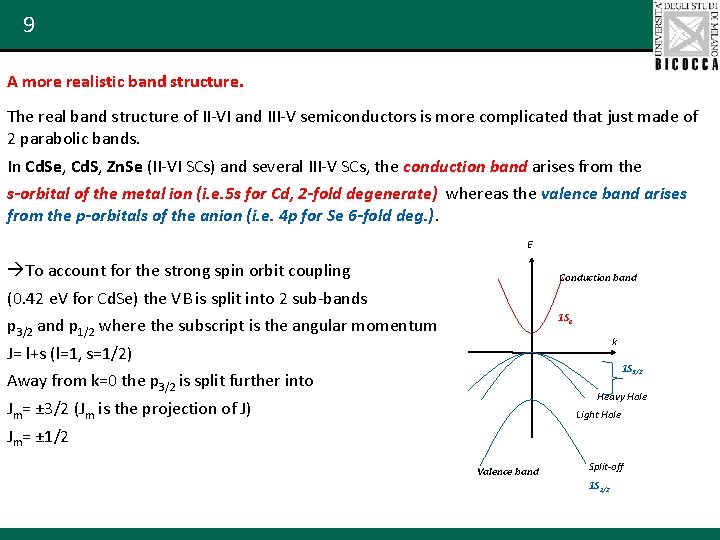
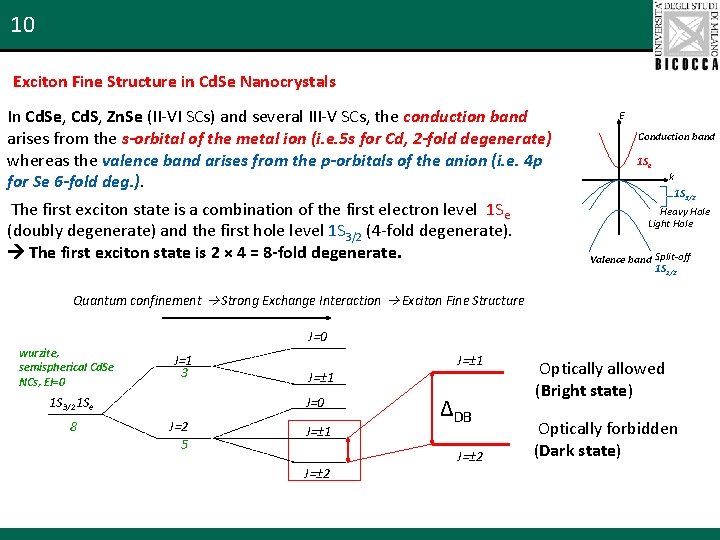
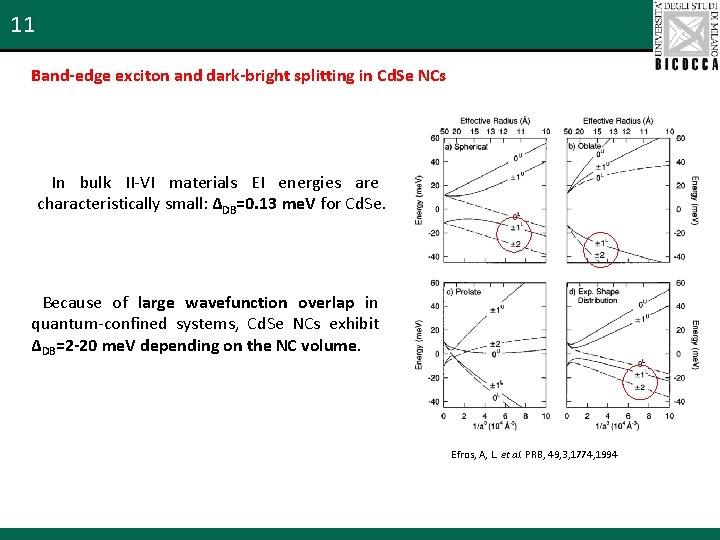
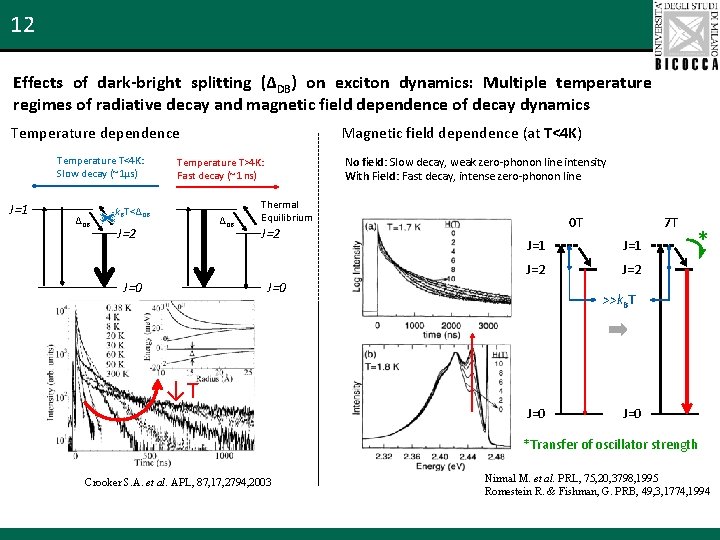
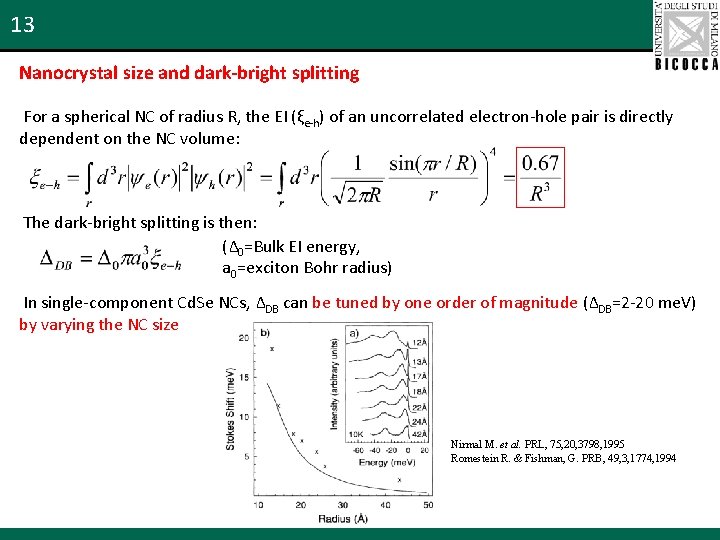
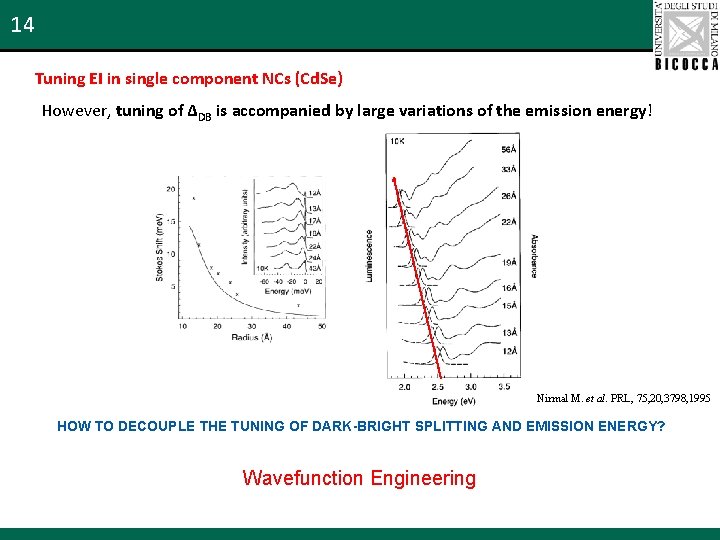
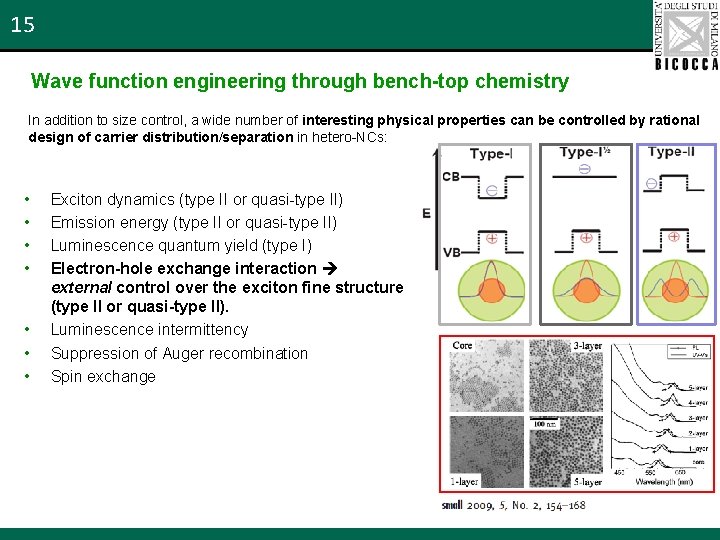
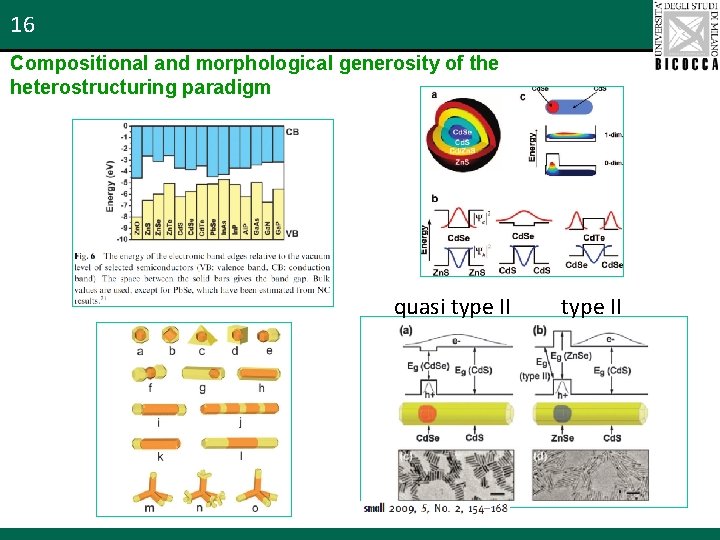
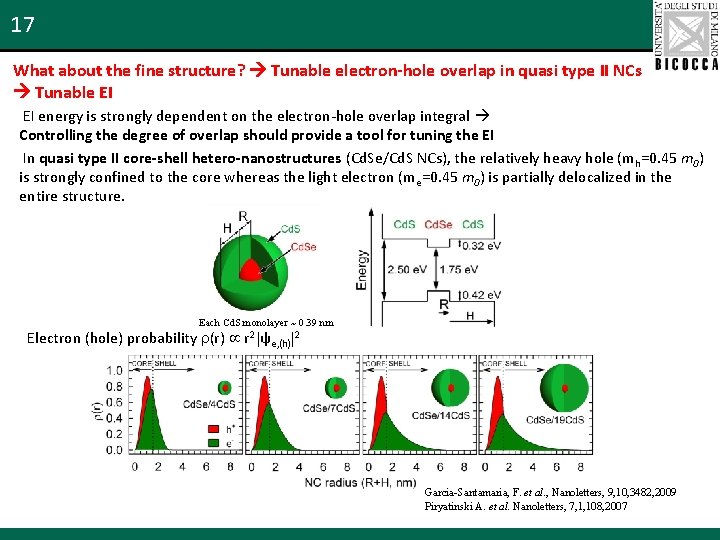
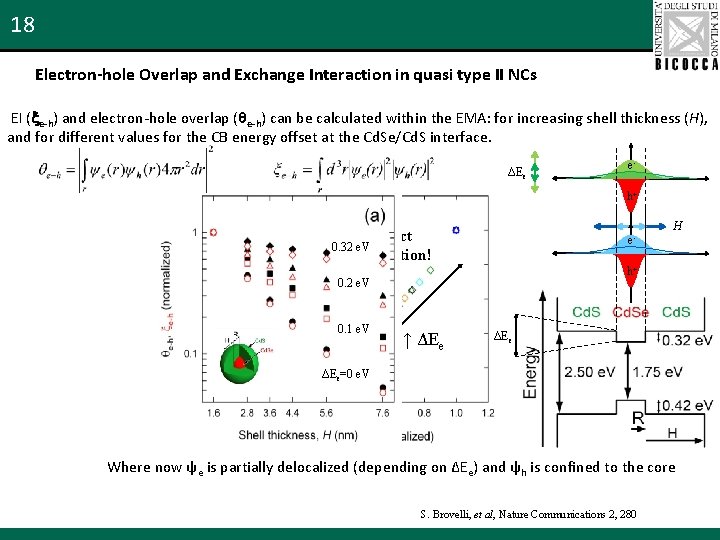
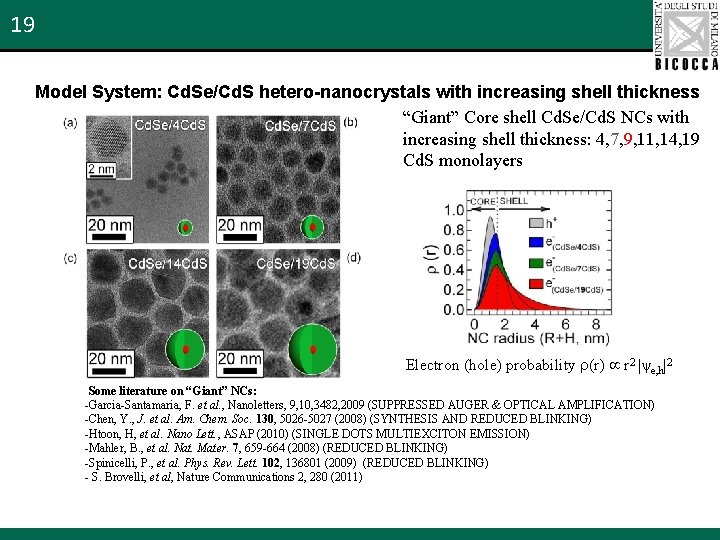
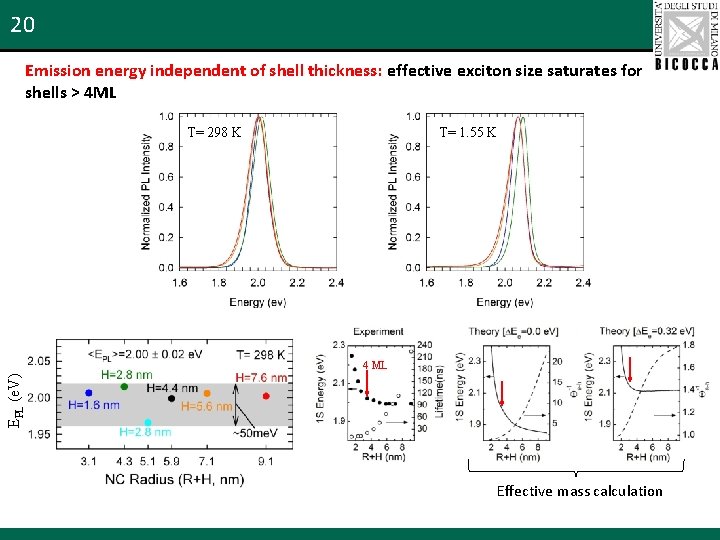
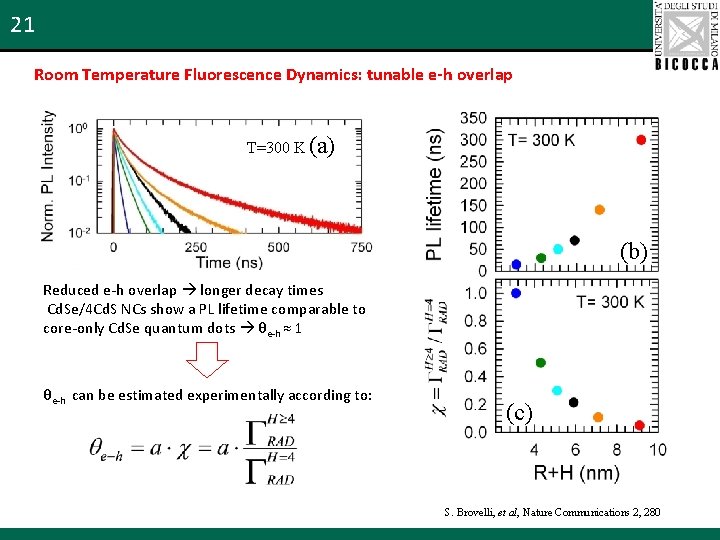
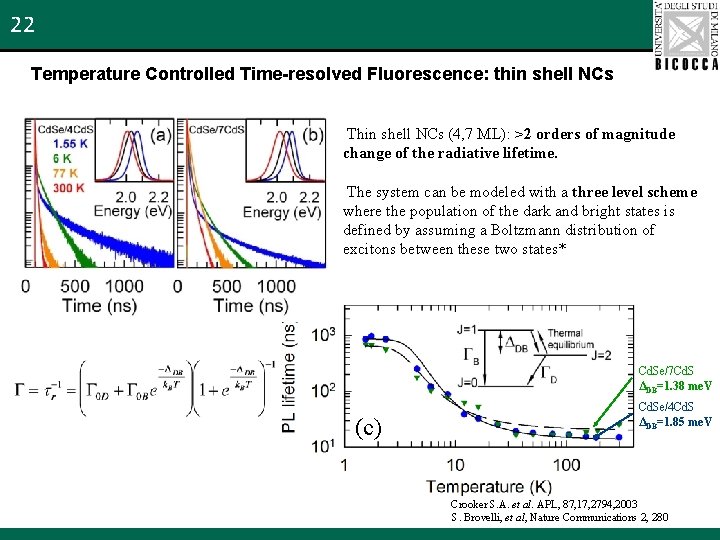
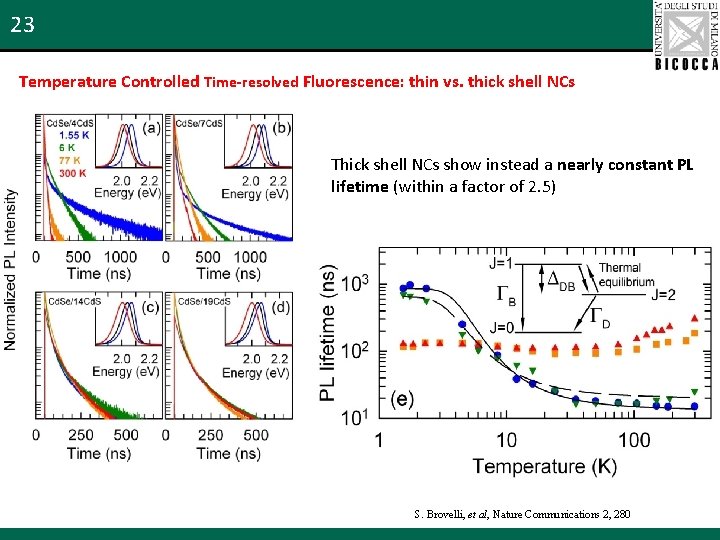
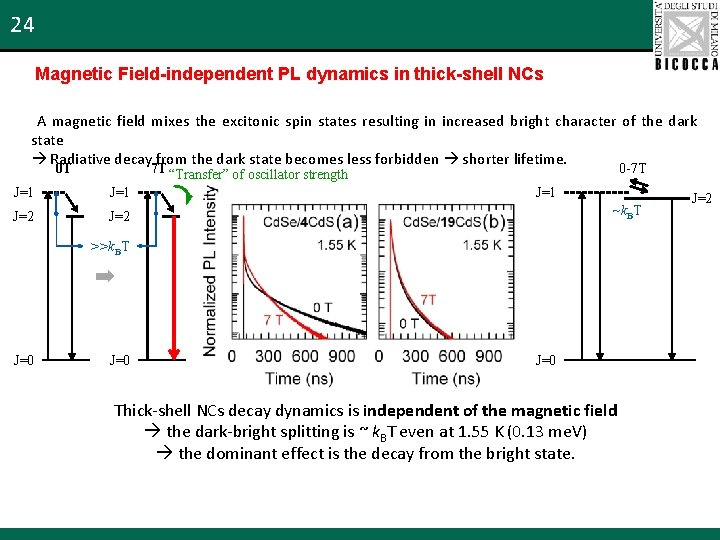
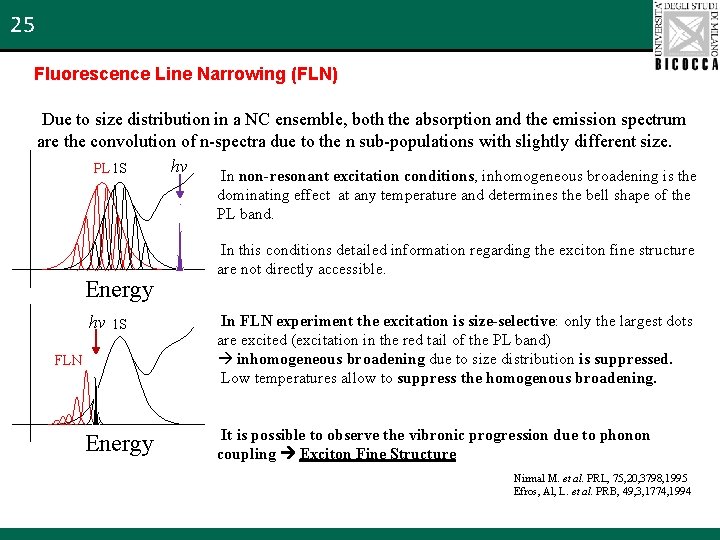
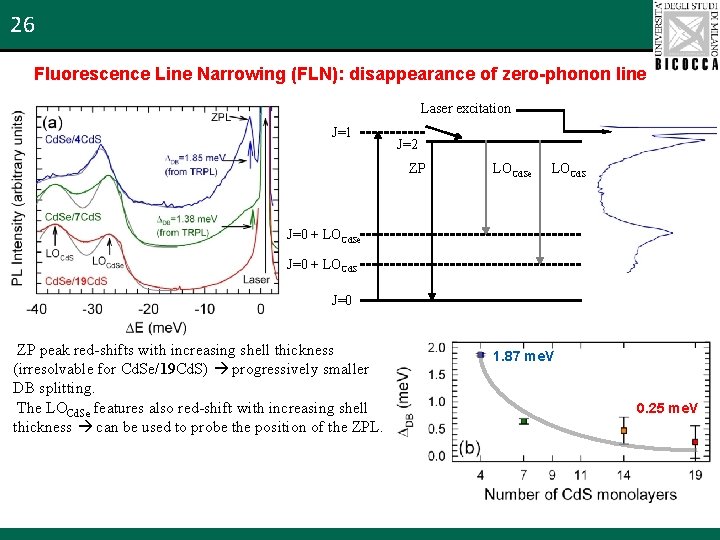
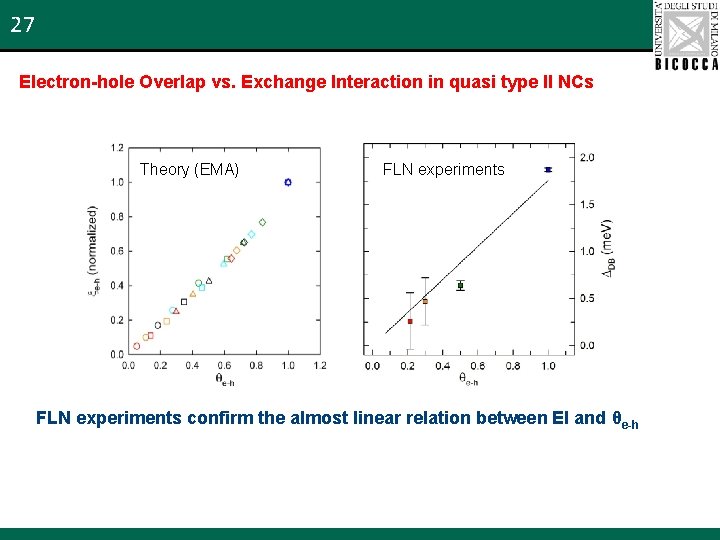
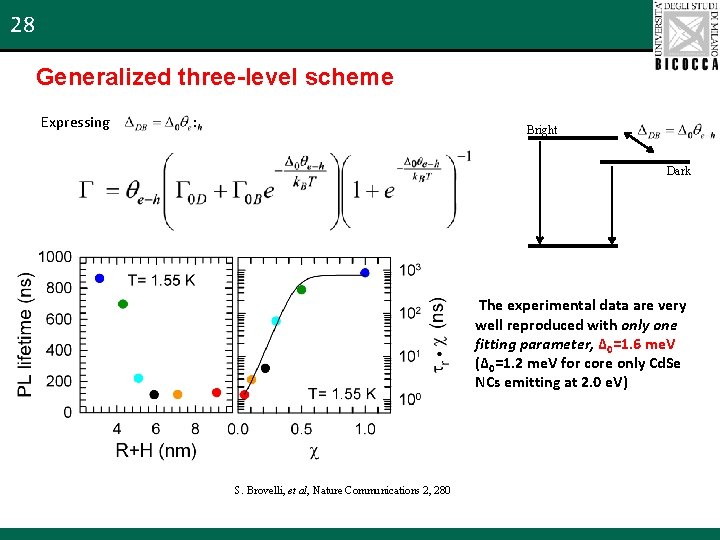
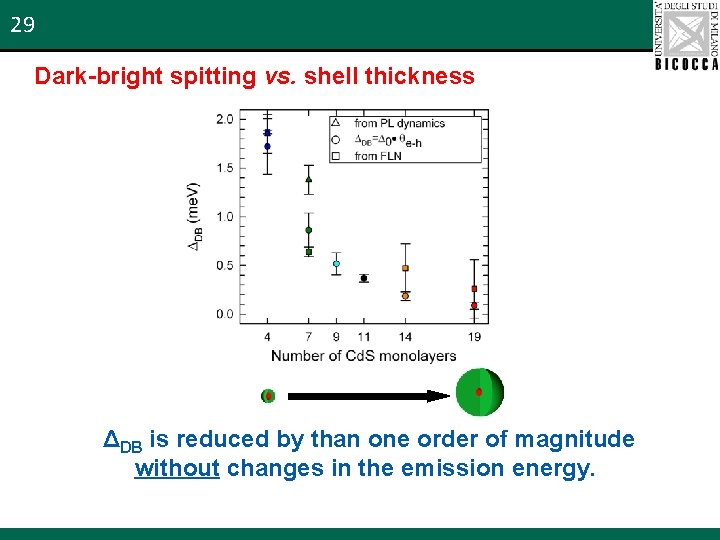
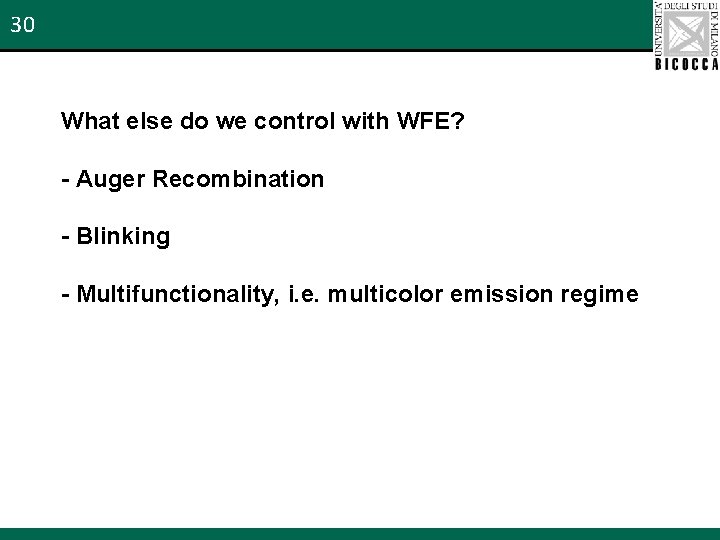
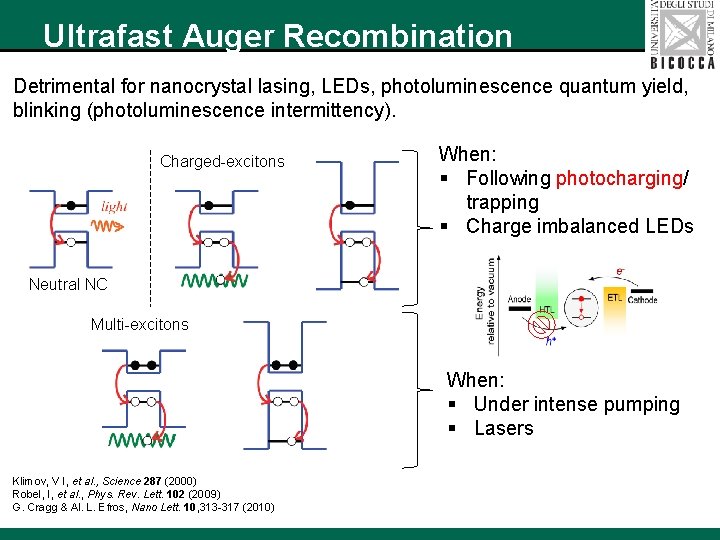
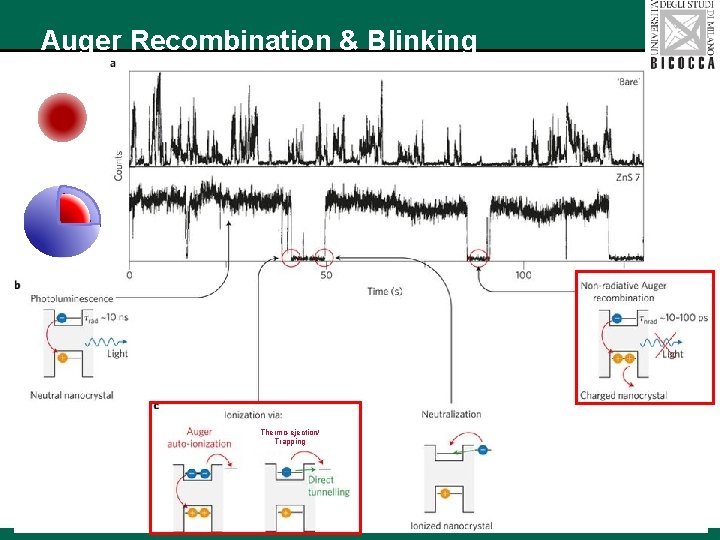
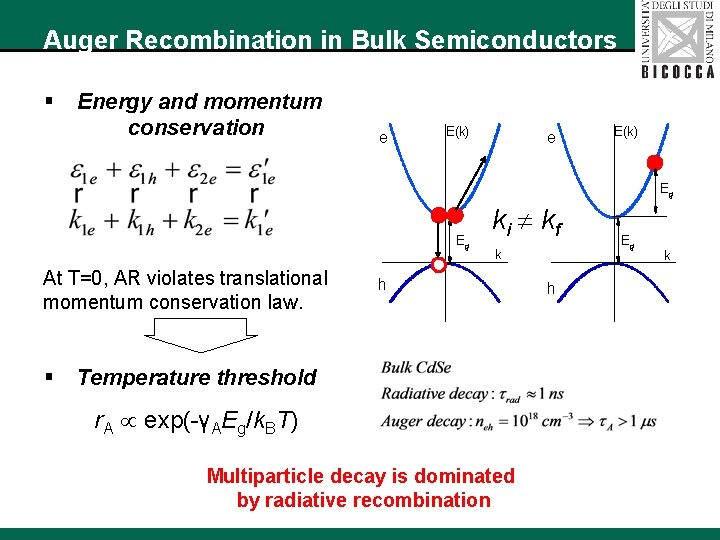
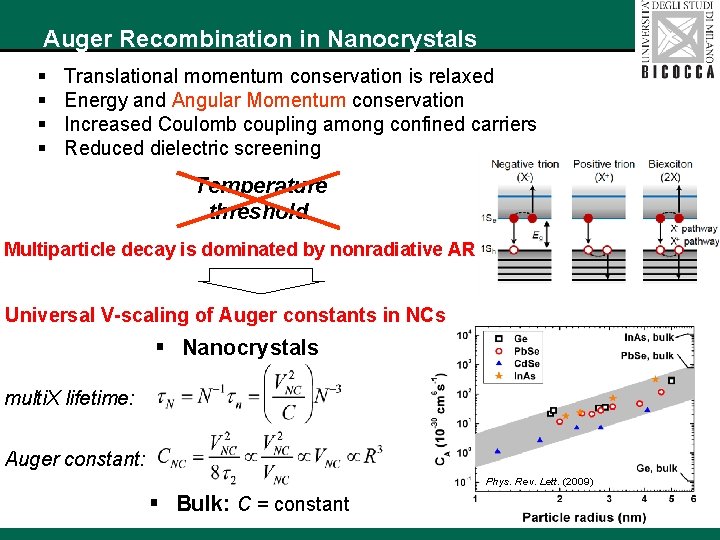
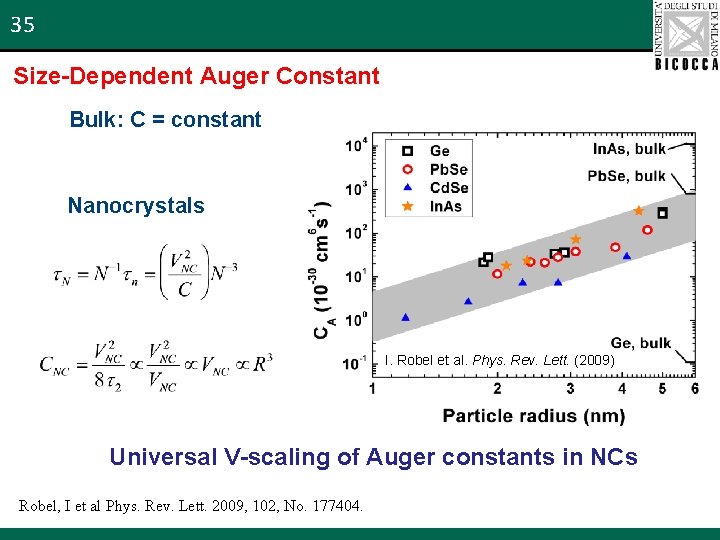
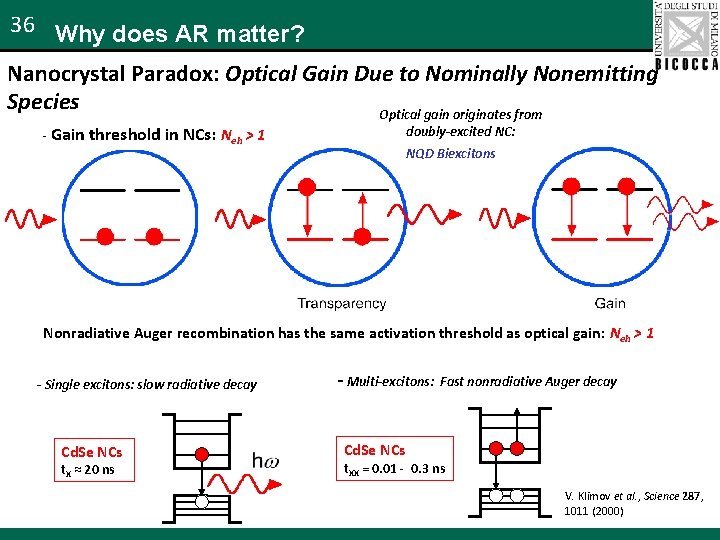
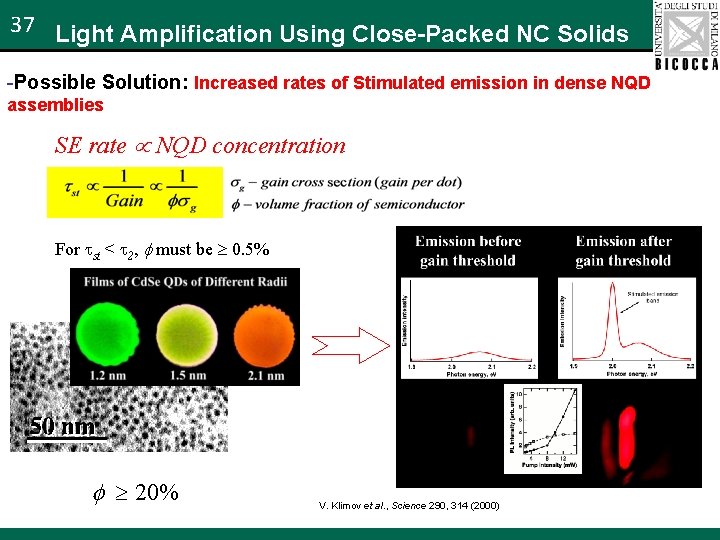
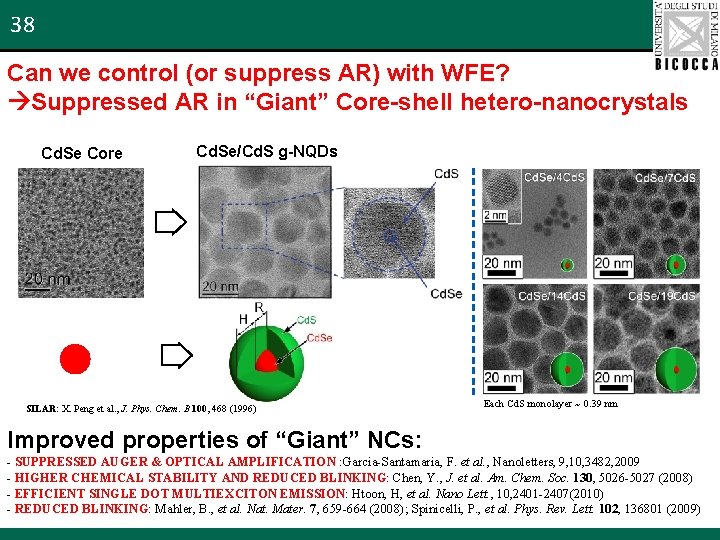
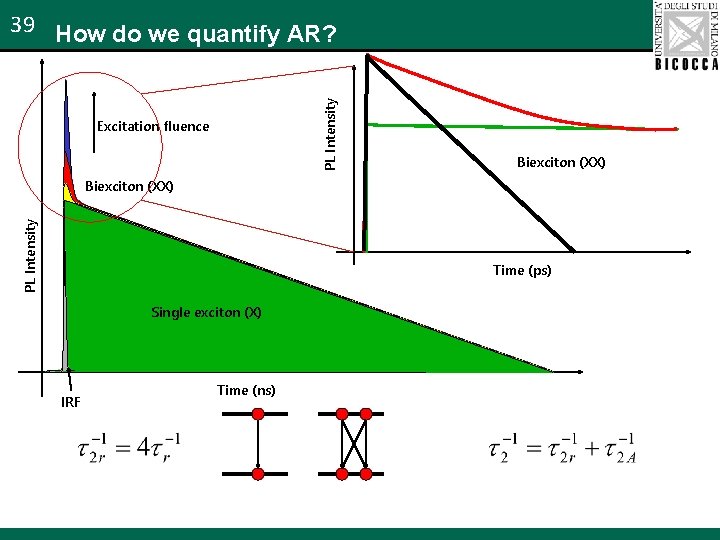
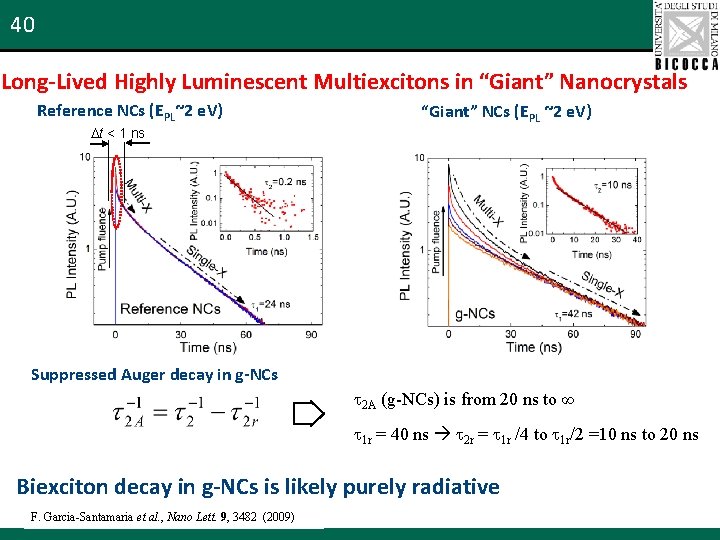
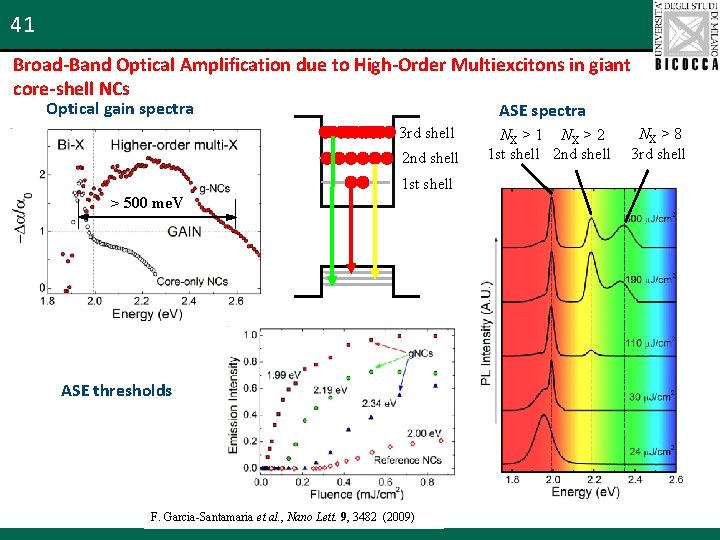
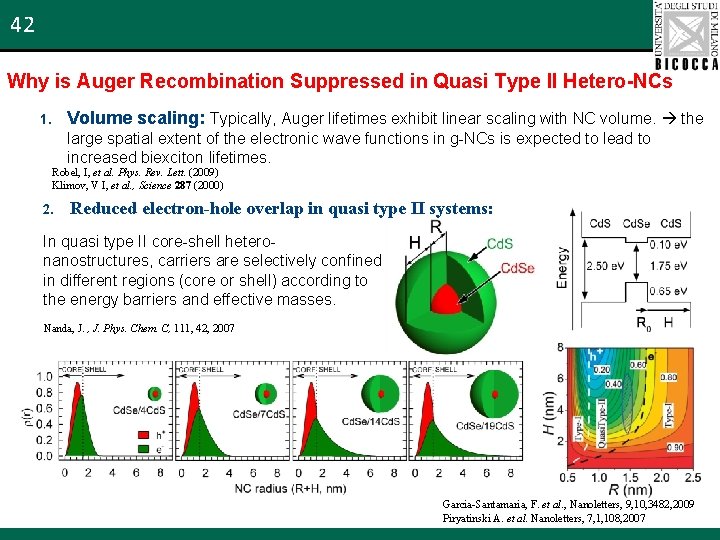
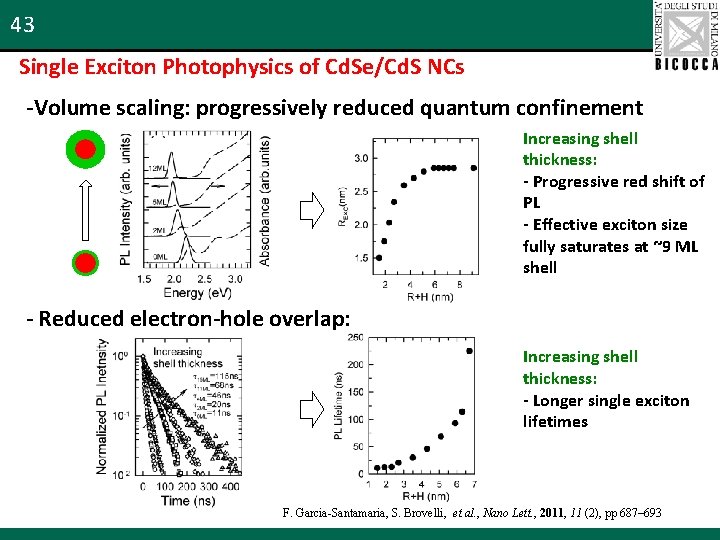
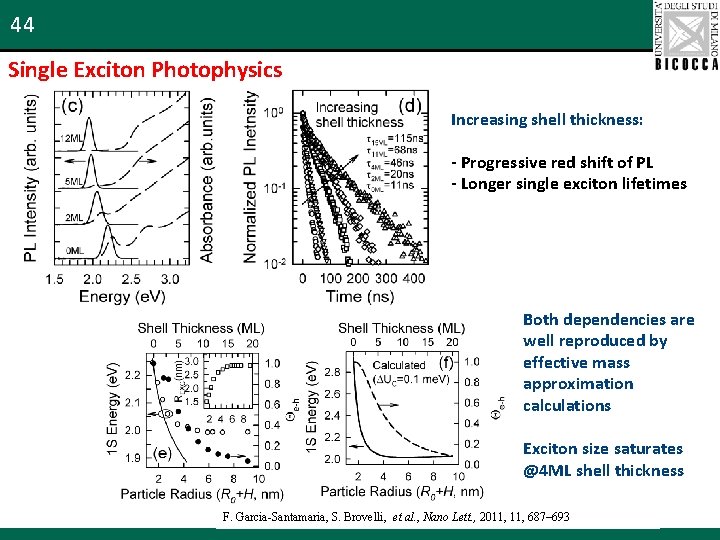
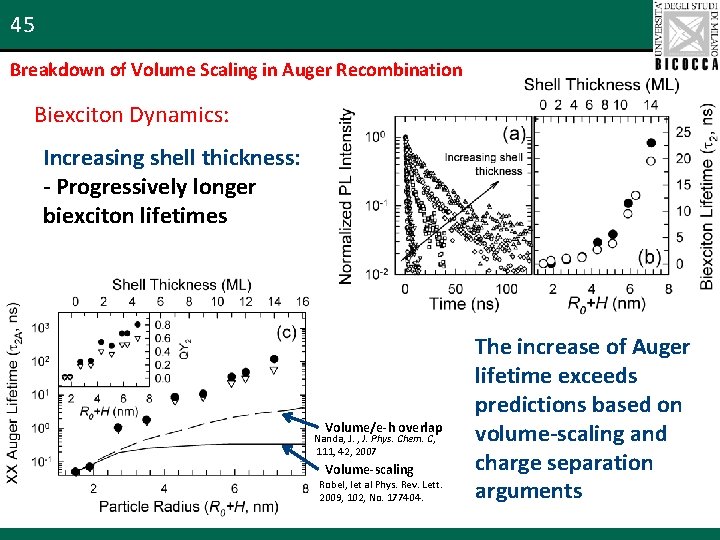
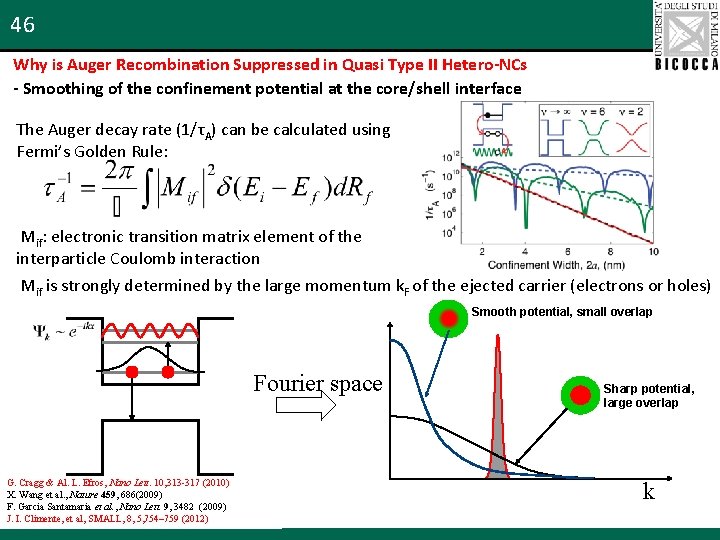
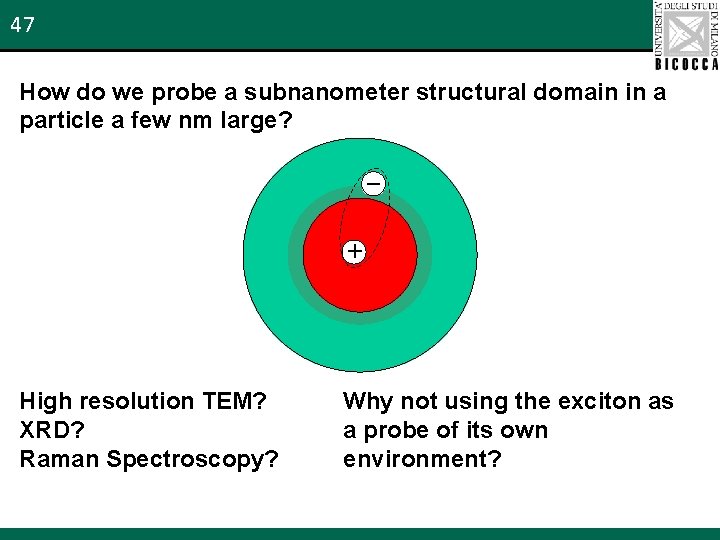
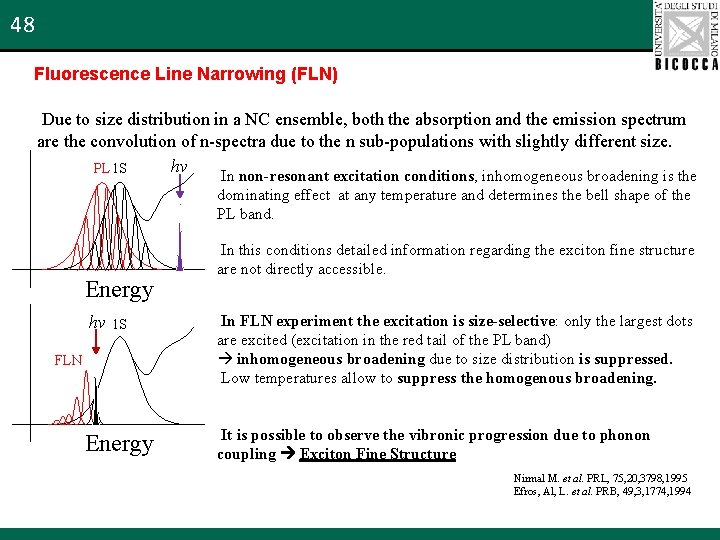
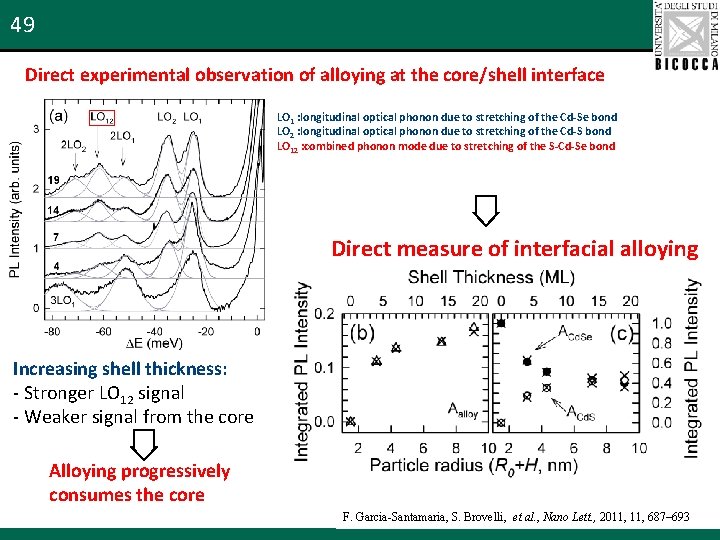
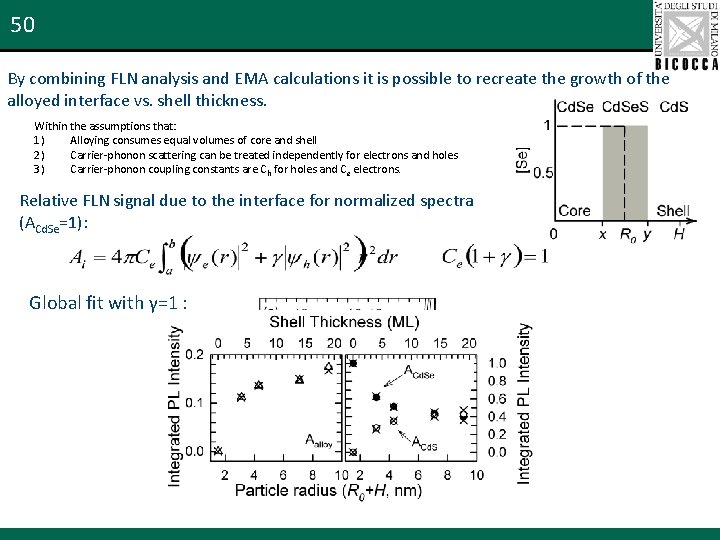
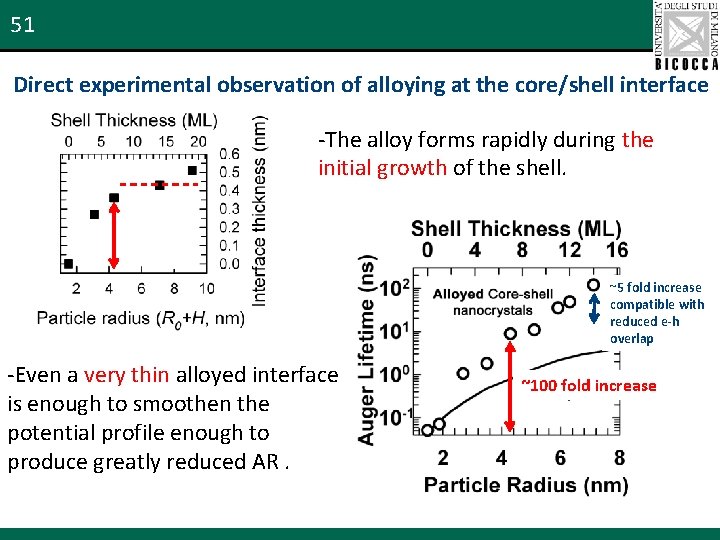
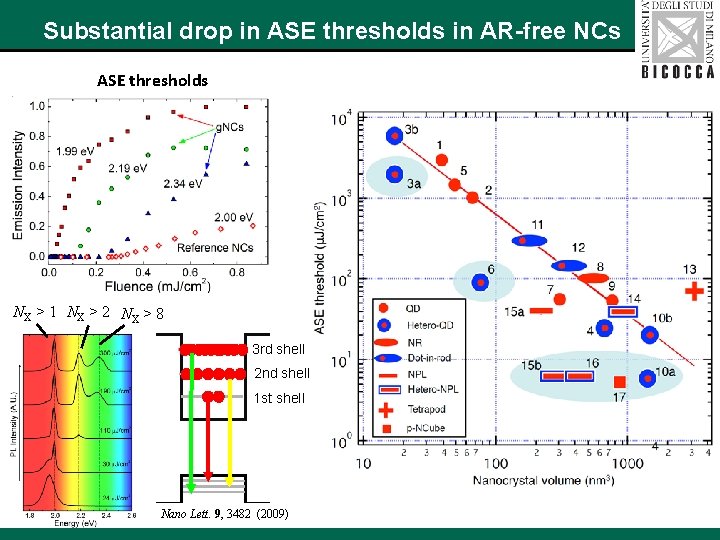
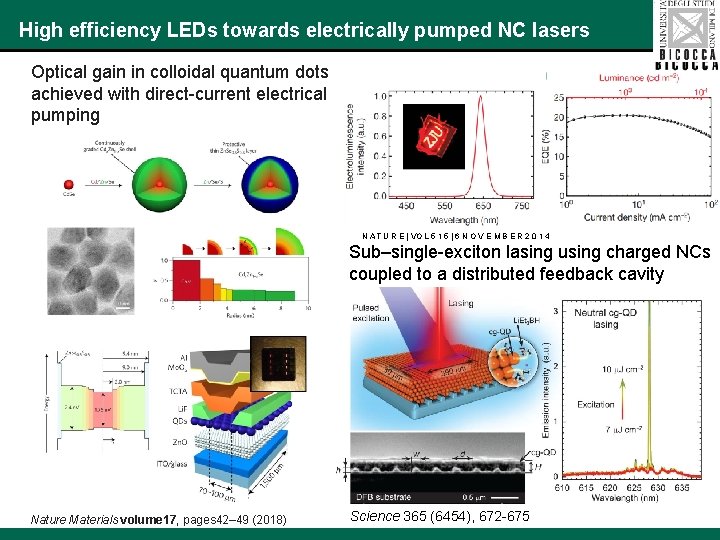
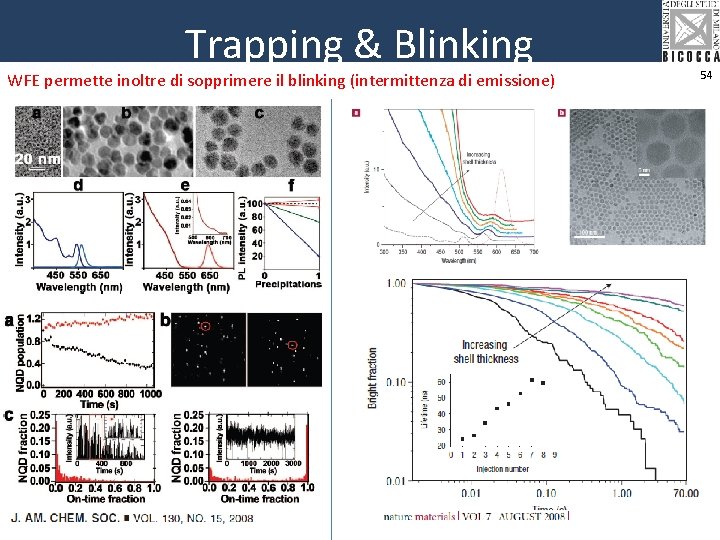
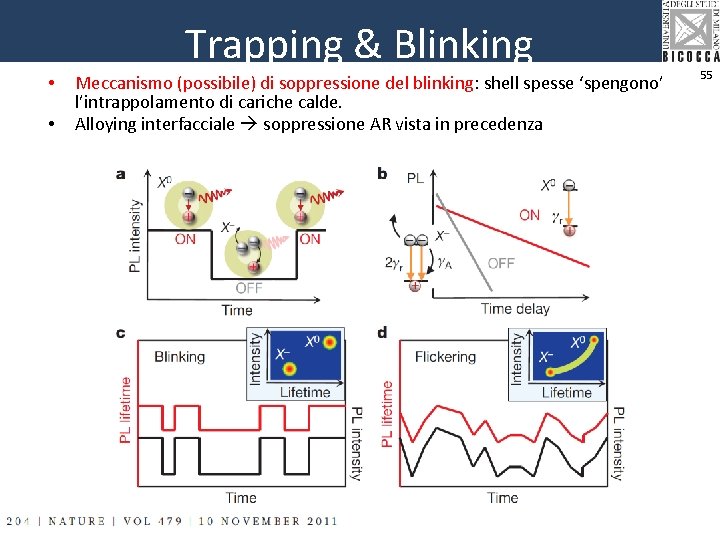
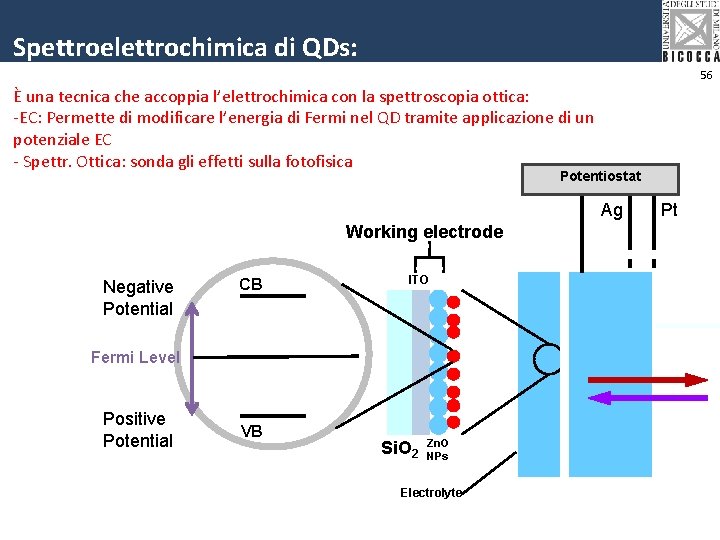
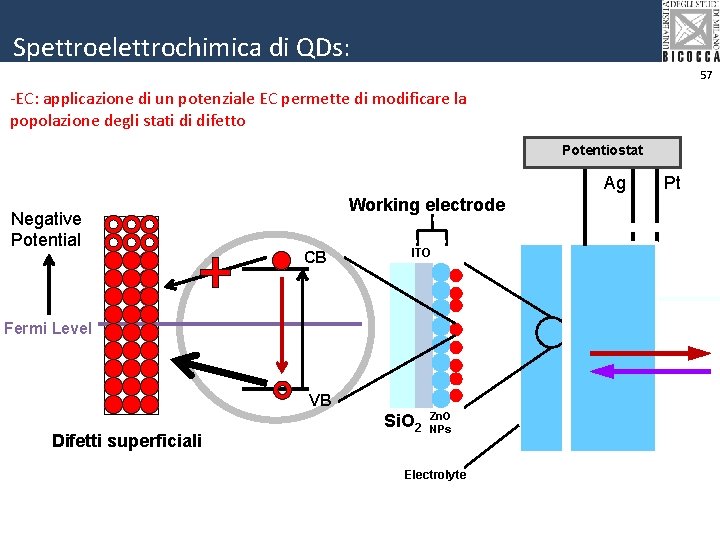
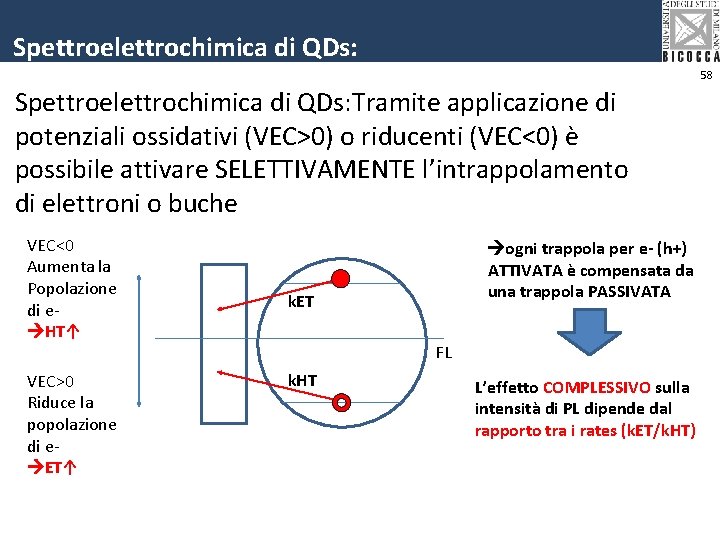
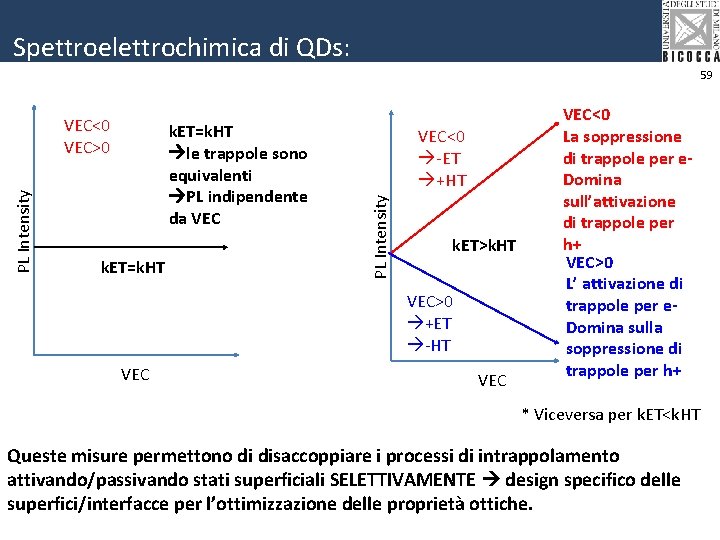
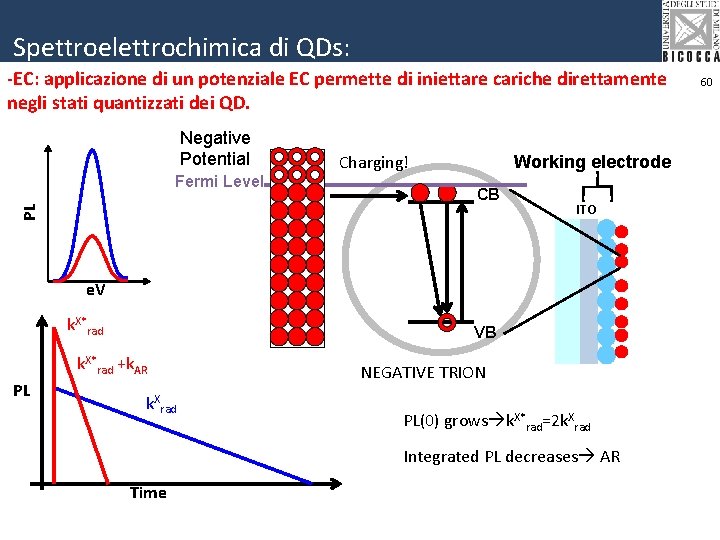
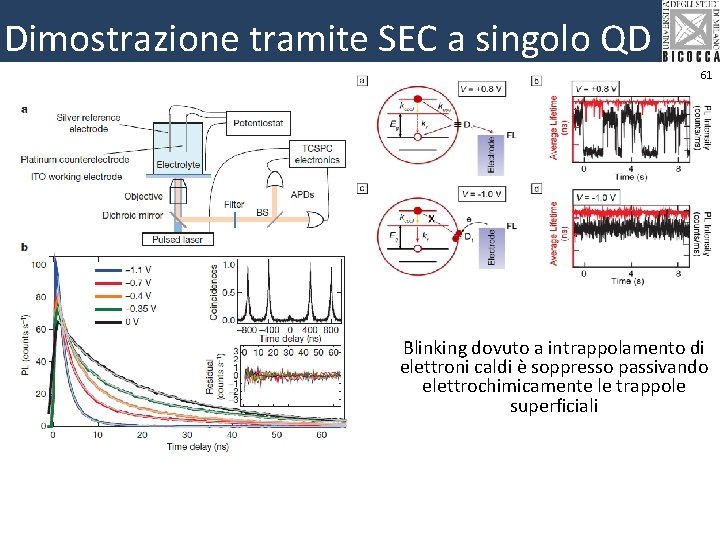
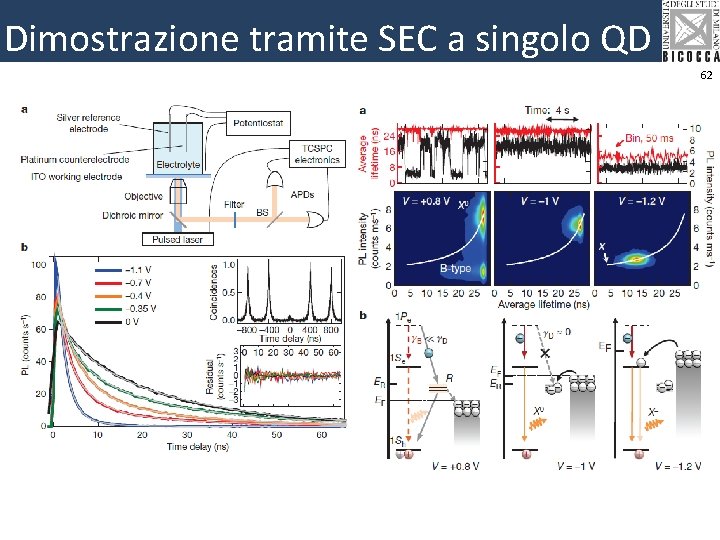
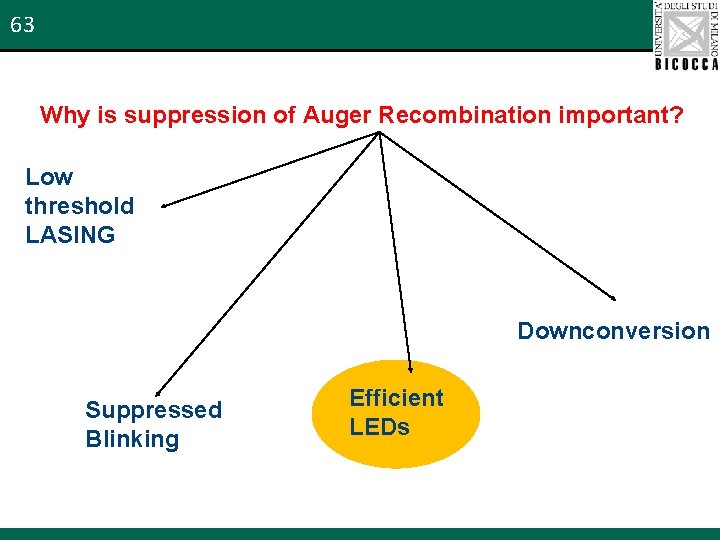
- Slides: 63
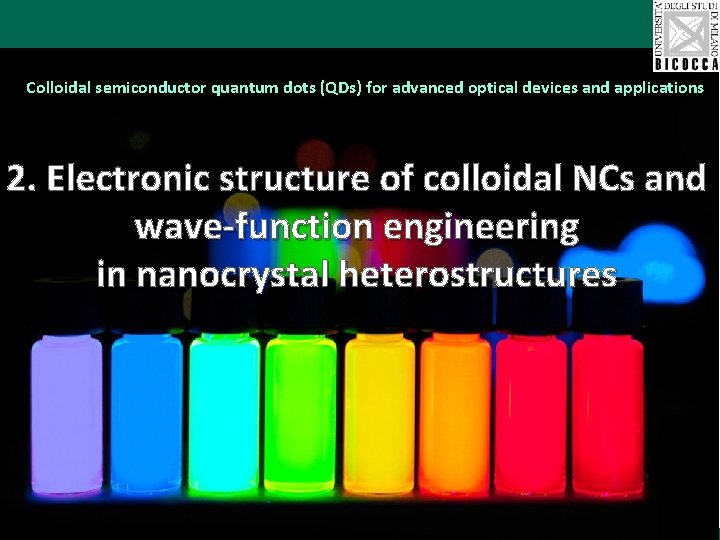
Colloidal semiconductor quantum dots (QDs) for advanced optical devices and applications 2. Electronic structure of colloidal NCs and wave-function engineering in nanocrystal heterostructures 1
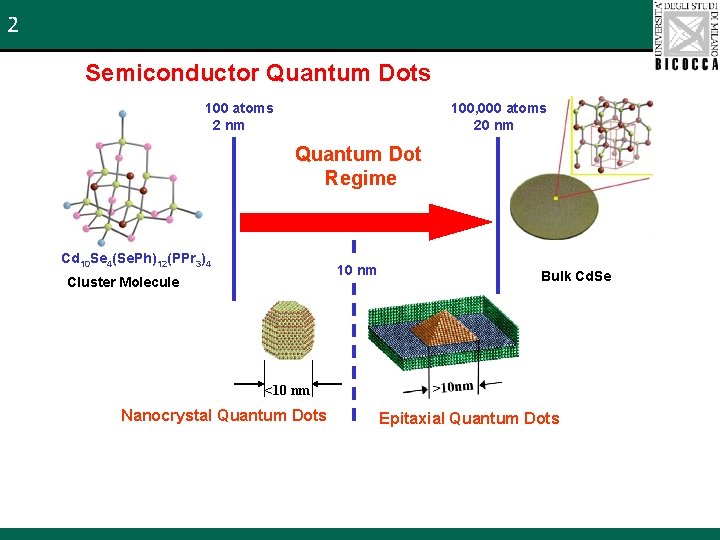
2 Semiconductor Quantum Dots 100 atoms 2 nm 100, 000 atoms 20 nm Quantum Dot Regime Cd 10 Se 4(Se. Ph)12(PPr 3)4 10 nm Cluster Molecule Bulk Cd. Se <10 nm Nanocrystal Quantum Dots Epitaxial Quantum Dots
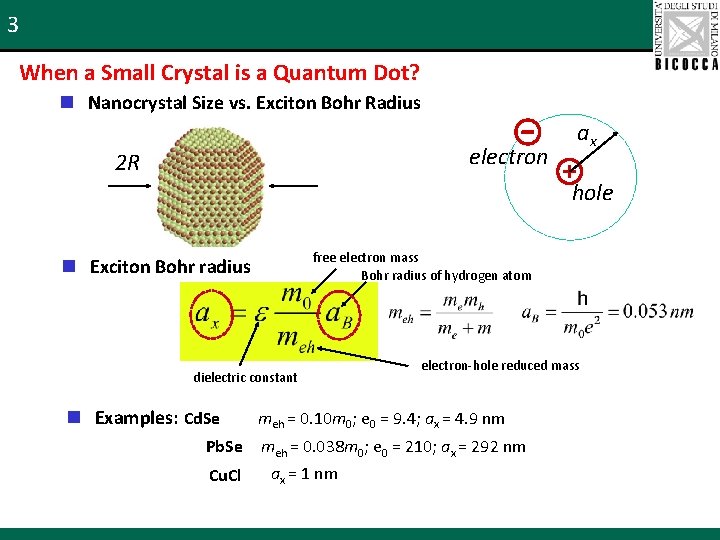
3 When a Small Crystal is a Quantum Dot? n Nanocrystal Size vs. Exciton Bohr Radius electron 2 R ax hole free electron mass Bohr radius of hydrogen atom n Exciton Bohr radius dielectric constant n Examples: Cd. Se Pb. Se Cu. Cl electron-hole reduced mass meh = 0. 10 m 0; e 0 = 9. 4; ax = 4. 9 nm meh = 0. 038 m 0; e 0 = 210; ax = 292 nm ax = 1 nm
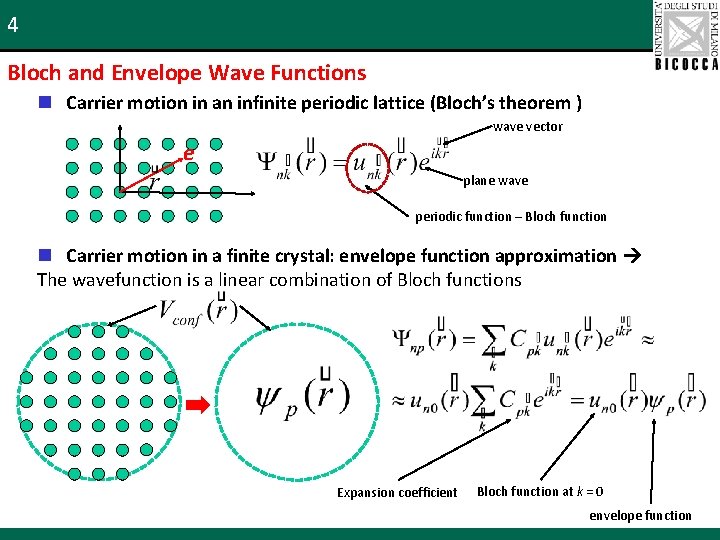
4 Bloch and Envelope Wave Functions n Carrier motion in an infinite periodic lattice (Bloch’s theorem ) wave vector e plane wave periodic function – Bloch function n Carrier motion in a finite crystal: envelope function approximation The wavefunction is a linear combination of Bloch functions Expansion coefficient Bloch function at k = 0 envelope function
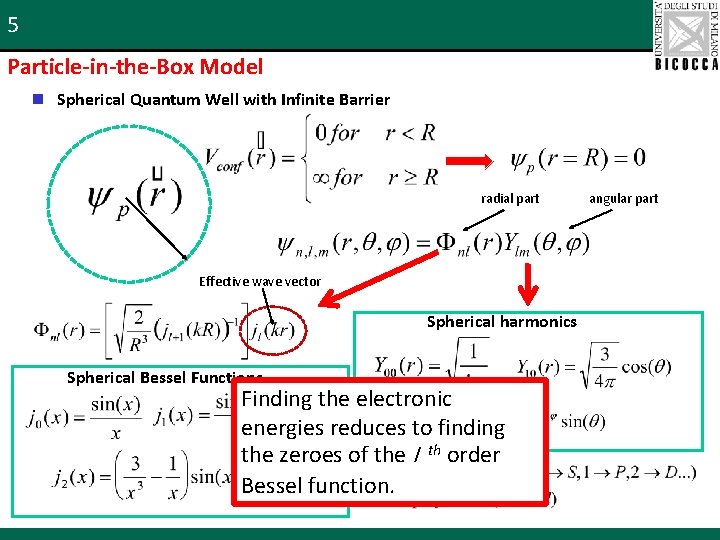
5 Particle-in-the-Box Model n Spherical Quantum Well with Infinite Barrier radial part Effective wave vector Spherical harmonics Spherical Bessel Functions Finding the electronic energies reduces to finding the zeroes of the l th order Bessel function. angular part
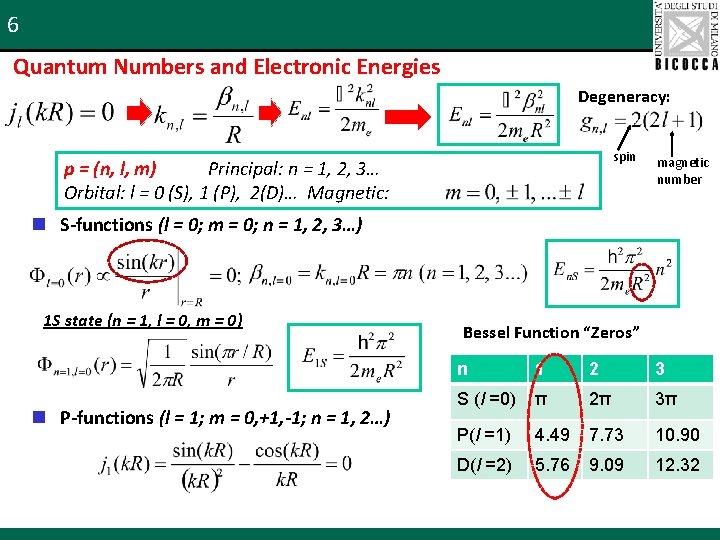
6 Quantum Numbers and Electronic Energies Degeneracy: spin p = (n, l, m) Principal: n = 1, 2, 3… Orbital: l = 0 (S), 1 (P), 2(D)… Magnetic: magnetic number n S-functions (l = 0; m = 0; n = 1, 2, 3…) 1 S state (n = 1, l = 0, m = 0) n P-functions (l = 1; m = 0, +1, -1; n = 1, 2…) Bessel Function “Zeros” n 1 2 3 S (l =0) π 2π 3π P(l =1) 4. 49 7. 73 10. 90 D(l =2) 5. 76 9. 09 12. 32
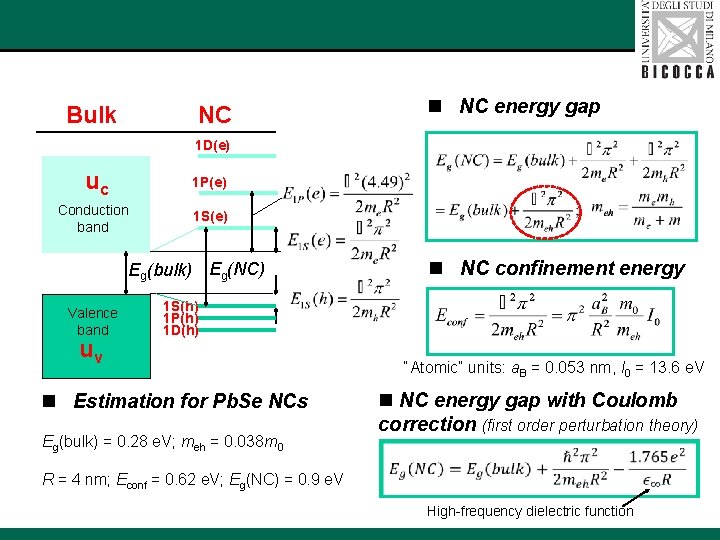
NC Bulk n NC energy gap 1 D(e) uc 1 P(e) Conduction band 1 S(e) Eg(bulk) Eg(NC) Valence band uv n NC confinement energy 1 S(h) 1 P(h) 1 D(h) n Estimation for Pb. Se NCs Eg(bulk) = 0. 28 e. V; meh = 0. 038 m 0 “Atomic” units: a. B = 0. 053 nm, I 0 = 13. 6 e. V n NC energy gap with Coulomb correction (first order perturbation theory) R = 4 nm; Econf = 0. 62 e. V; Eg(NC) = 0. 9 e. V High-frequency dielectric function
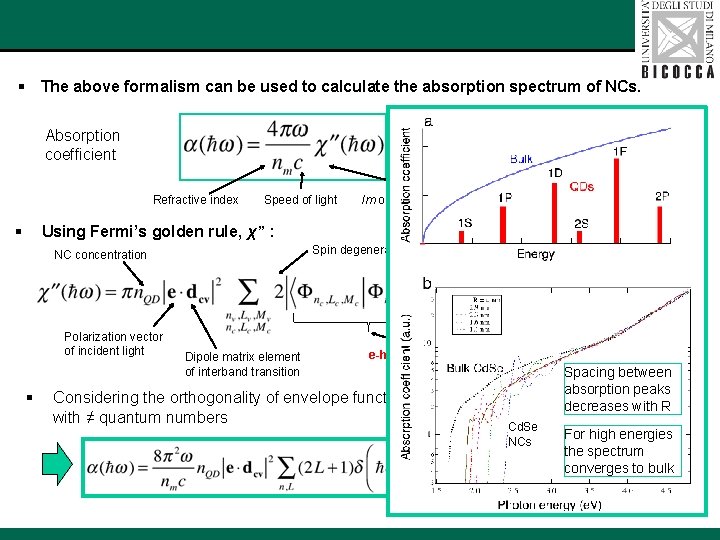
§ The above formalism can be used to calculate the absorption spectrum of NCs. Absorption coefficient Refractive index § Speed of light Im optical susceptibility Using Fermi’s golden rule, χ” : Polarization vector of incident light § VB, CB energies Spin degeneracy NC concentration Dipole matrix element of interband transition e-h overlap integral Considering the orthogonality of envelope functions with ≠ quantum numbers Spacing between absorption peaks decreases with R Cd. Se NCs For high energies the spectrum converges to bulk
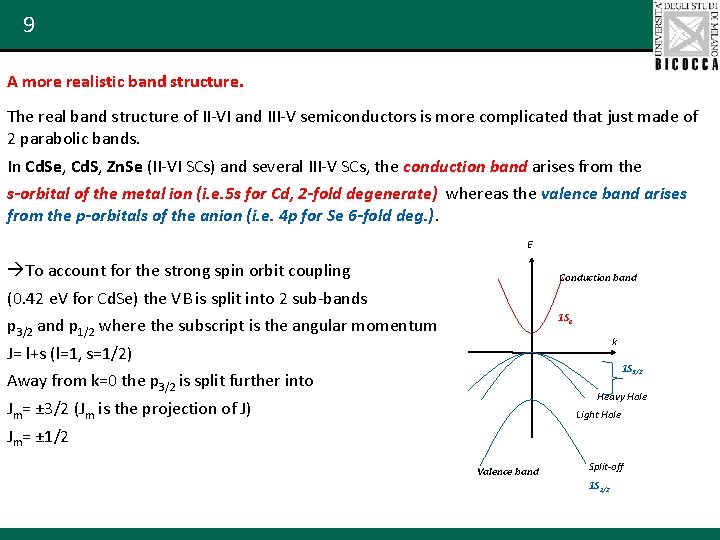
9 A more realistic band structure. The real band structure of II-VI and III-V semiconductors is more complicated that just made of 2 parabolic bands. In Cd. Se, Cd. S, Zn. Se (II-VI SCs) and several III-V SCs, the conduction band arises from the s-orbital of the metal ion (i. e. 5 s for Cd, 2 -fold degenerate) whereas the valence band arises from the p-orbitals of the anion (i. e. 4 p for Se 6 -fold deg. ). E To account for the strong spin orbit coupling (0. 42 e. V for Cd. Se) the VB is split into 2 sub-bands p 3/2 and p 1/2 where the subscript is the angular momentum J= l+s (l=1, s=1/2) Away from k=0 the p 3/2 is split further into Jm= ± 3/2 (Jm is the projection of J) Jm= ± 1/2 Conduction band 1 Se k 1 S 3/2 Heavy Hole Light Hole Valence band Split-off 1 S 1/2
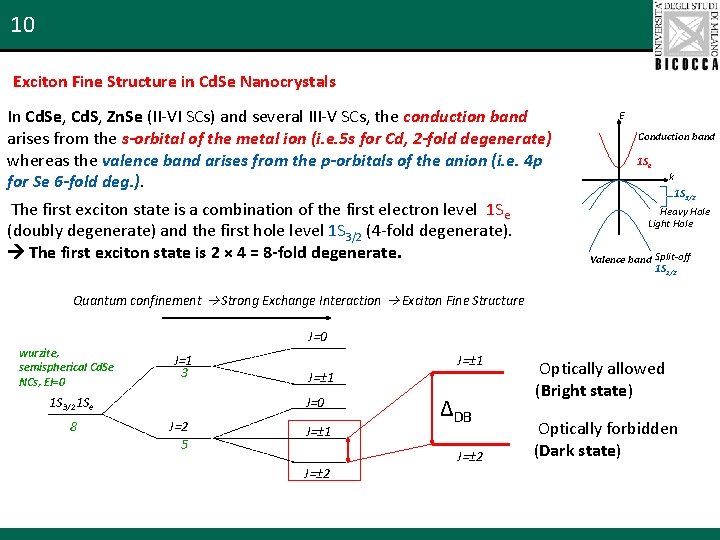
10 Exciton Fine Structure in Cd. Se Nanocrystals In Cd. Se, Cd. S, Zn. Se (II-VI SCs) and several III-V SCs, the conduction band arises from the s-orbital of the metal ion (i. e. 5 s for Cd, 2 -fold degenerate) whereas the valence band arises from the p-orbitals of the anion (i. e. 4 p for Se 6 -fold deg. ). The first exciton state is a combination of the first electron level 1 Se (doubly degenerate) and the first hole level 1 S 3/2 (4 -fold degenerate). The first exciton state is 2 × 4 = 8 -fold degenerate. E Conduction band 1 Se k 1 S 3/2 Heavy Hole Light Hole Valence band Split-off 1 S 1/2 Quantum confinement Strong Exchange Interaction Exciton Fine Structure J=0 wurzite, semispherical Cd. Se NCs, EI=0 J=1 3 1 S 3/21 Se 8 J=± 1 J=0 J=2 5 J=± 1 ΔDB J=± 2 Optically allowed (Bright state) Optically forbidden (Dark state)
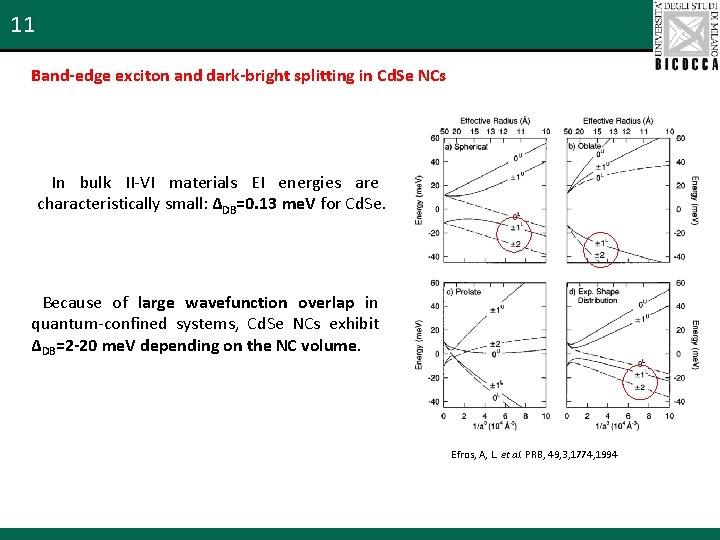
11 Band-edge exciton and dark-bright splitting in Cd. Se NCs In bulk II-VI materials EI energies are characteristically small: ΔDB=0. 13 me. V for Cd. Se. Because of large wavefunction overlap in quantum-confined systems, Cd. Se NCs exhibit ΔDB=2 -20 me. V depending on the NC volume. Efros, A, L. et al. PRB, 49, 3, 1774, 1994
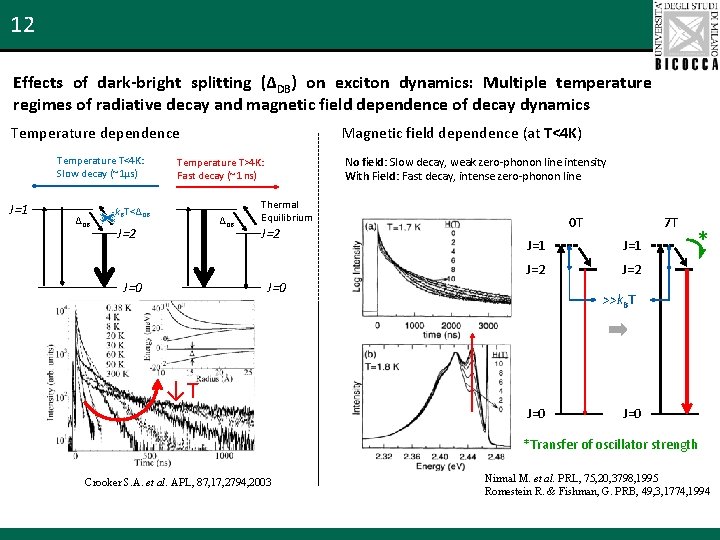
12 Effects of dark-bright splitting (ΔDB) on exciton dynamics: Multiple temperature regimes of radiative decay and magnetic field dependence of decay dynamics Magnetic field dependence (at T<4 K) Temperature dependence Temperature T<4 K: Slow decay (~1μs) J=1 ΔDB No field: Slow decay, weak zero-phonon line intensity With Field: Fast decay, intense zero-phonon line Temperature T>4 K: Fast decay (~1 ns) k. BT<ΔDB J=2 J=0 Thermal Equilibrium J=2 0 T 7 T J=1 J=2 J=0 * >>k. BT ↓T J=0 *Transfer of oscillator strength Crooker S. A. et al. APL, 87, 17, 2794, 2003 Nirmal M. et al. PRL, 75, 20, 3798, 1995 Romestein R. & Fishman, G. PRB, 49, 3, 1774, 1994
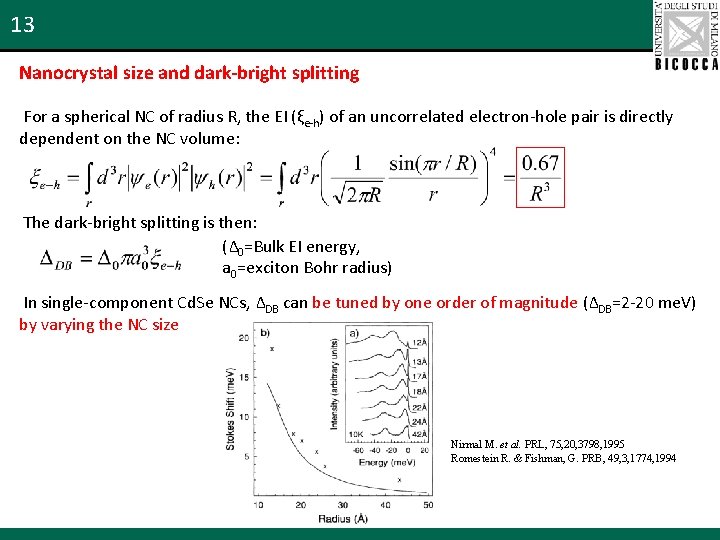
13 Nanocrystal size and dark-bright splitting For a spherical NC of radius R, the EI (ξe-h) of an uncorrelated electron-hole pair is directly dependent on the NC volume: The dark-bright splitting is then: (Δ 0=Bulk EI energy, a 0=exciton Bohr radius) In single-component Cd. Se NCs, ΔDB can be tuned by one order of magnitude (ΔDB=2 -20 me. V) by varying the NC size Nirmal M. et al. PRL, 75, 20, 3798, 1995 Romestein R. & Fishman, G. PRB, 49, 3, 1774, 1994
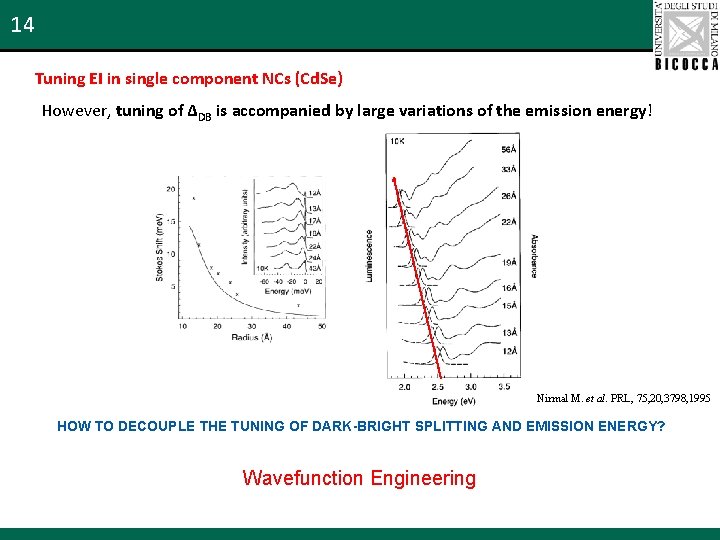
14 Tuning EI in single component NCs (Cd. Se) However, tuning of ΔDB is accompanied by large variations of the emission energy! Nirmal M. et al. PRL, 75, 20, 3798, 1995 HOW TO DECOUPLE THE TUNING OF DARK-BRIGHT SPLITTING AND EMISSION ENERGY? Wavefunction Engineering
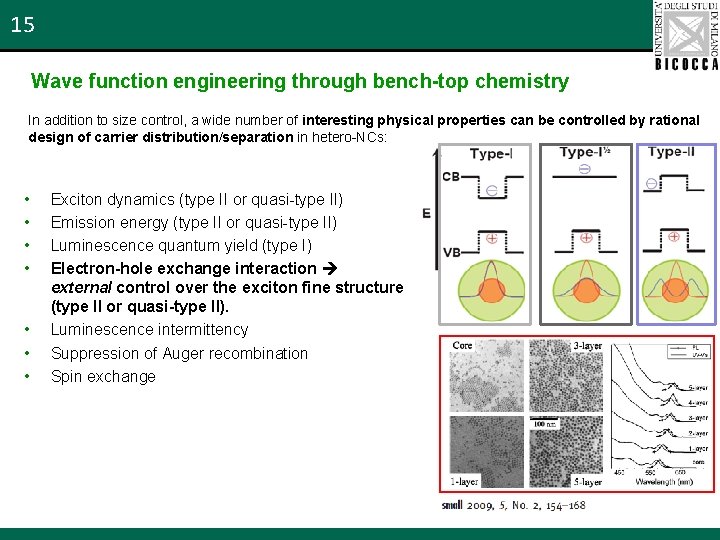
15 Wave function engineering through bench-top chemistry In addition to size control, a wide number of interesting physical properties can be controlled by rational design of carrier distribution/separation in hetero-NCs: • • Exciton dynamics (type II or quasi-type II) Emission energy (type II or quasi-type II) Luminescence quantum yield (type I) Electron-hole exchange interaction external control over the exciton fine structure (type II or quasi-type II). Luminescence intermittency Suppression of Auger recombination Spin exchange
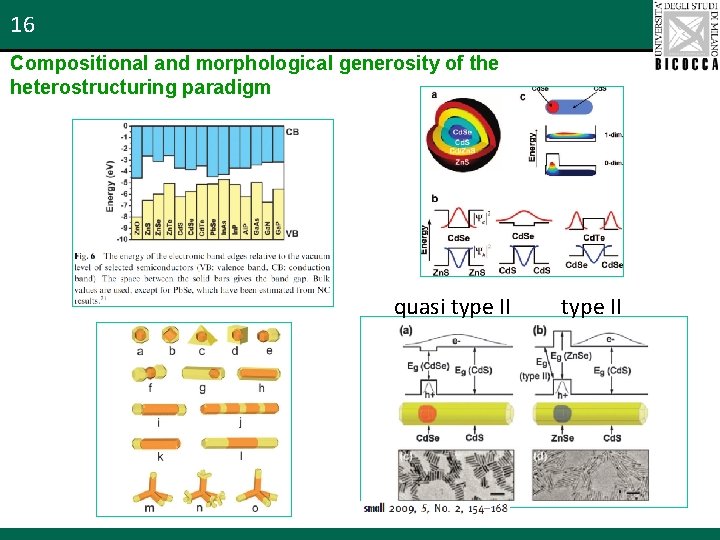
16 Compositional and morphological generosity of the heterostructuring paradigm quasi type II
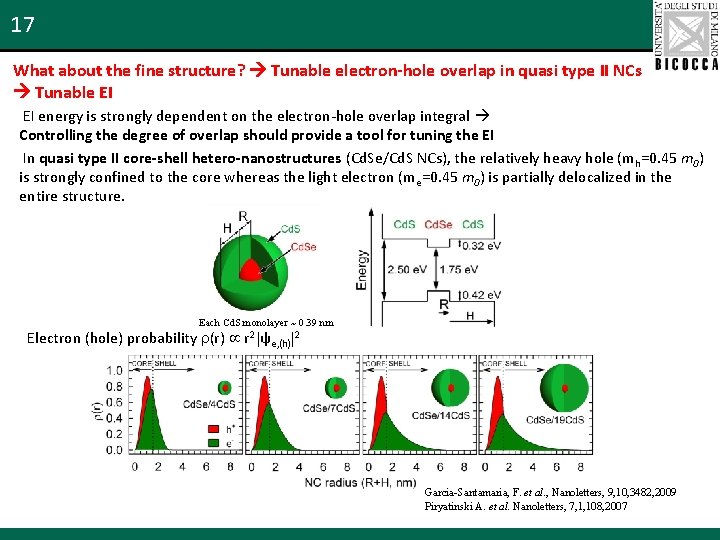
17 What about the fine structure? Tunable electron-hole overlap in quasi type II NCs Tunable EI EI energy is strongly dependent on the electron-hole overlap integral Controlling the degree of overlap should provide a tool for tuning the EI In quasi type II core-shell hetero-nanostructures (Cd. Se/Cd. S NCs), the relatively heavy hole (mh=0. 45 m 0) is strongly confined to the core whereas the light electron (me=0. 45 m 0) is partially delocalized in the entire structure. Electron (hole) Each Cd. S monolayer ~ 0. 39 nm probability (r) r 2 ψe, (h) 2 Garcia-Santamaria, F. et al. , Nanoletters, 9, 10, 3482, 2009 Piryatinski A. et al. Nanoletters, 7, 1, 108, 2007
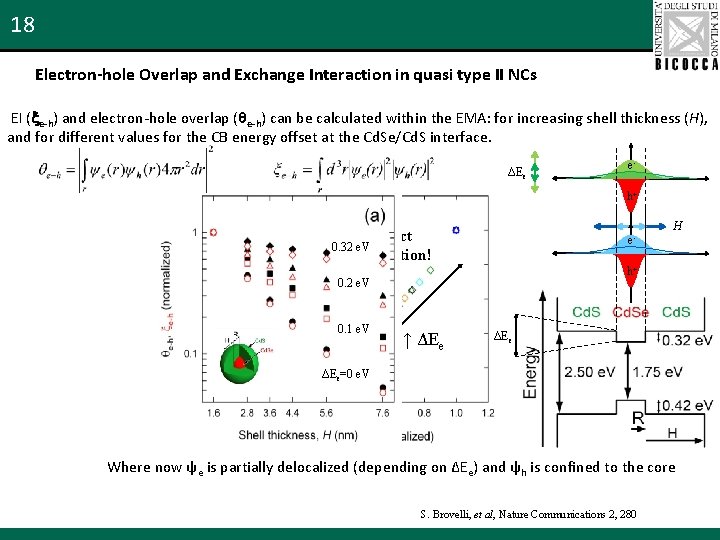
18 Electron-hole Overlap and Exchange Interaction in quasi type II NCs EI ( e-h) and electron-hole overlap (θe-h) can be calculated within the EMA: for increasing shell thickness (H), and for different values for the CB energy offset at the Cd. Se/Cd. S interface. ΔEe eh+ H Almost perfect 0. 32 e. V LINEAR relation! eh+ 0. 2 e. V 0. 1 e. V ↑ ΔEe ΔEe=0 e. V Where now ψe is partially delocalized (depending on ΔEe) and ψh is confined to the core S. Brovelli, et al, Nature Communications 2, 280
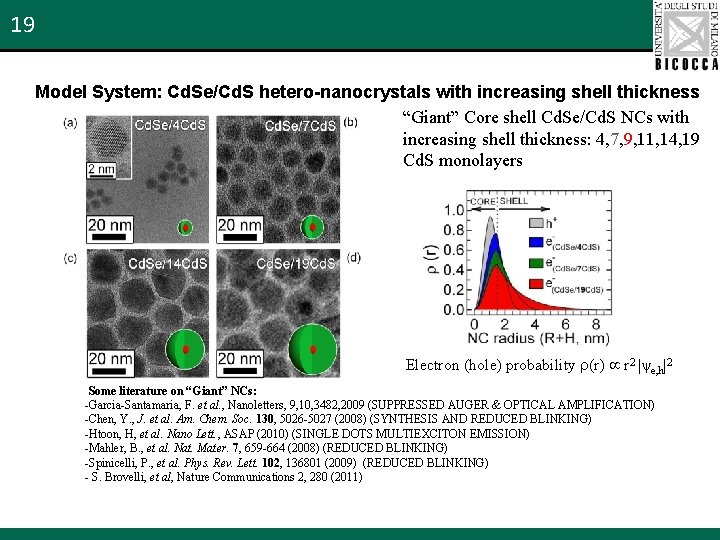
19 Model System: Cd. Se/Cd. S hetero-nanocrystals with increasing shell thickness “Giant” Core shell Cd. Se/Cd. S NCs with increasing shell thickness: 4, 7, 9, 11, 14, 19 Cd. S monolayers Electron (hole) probability (r) r 2 ψe, h 2 Some literature on “Giant” NCs: -Garcia-Santamaria, F. et al. , Nanoletters, 9, 10, 3482, 2009 (SUPPRESSED AUGER & OPTICAL AMPLIFICATION) -Chen, Y. , J. et al. Am. Chem. Soc. 130, 5026 -5027 (2008) (SYNTHESIS AND REDUCED BLINKING) -Htoon, H, et al. Nano Lett. , ASAP (2010) (SINGLE DOTS MULTIEXCITON EMISSION) -Mahler, B. , et al. Nat. Mater. 7, 659 -664 (2008) (REDUCED BLINKING) -Spinicelli, P. , et al. Phys. Rev. Lett. 102, 136801 (2009) (REDUCED BLINKING) - S. Brovelli, et al, Nature Communications 2, 280 (2011)
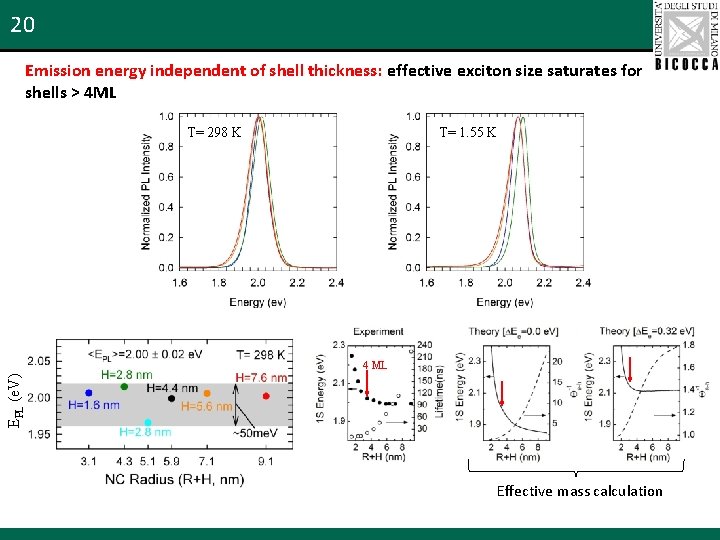
20 Emission energy independent of shell thickness: effective exciton size saturates for shells > 4 ML T= 298 K T= 1. 55 K EPL (e. V) 4 ML Effective mass calculation
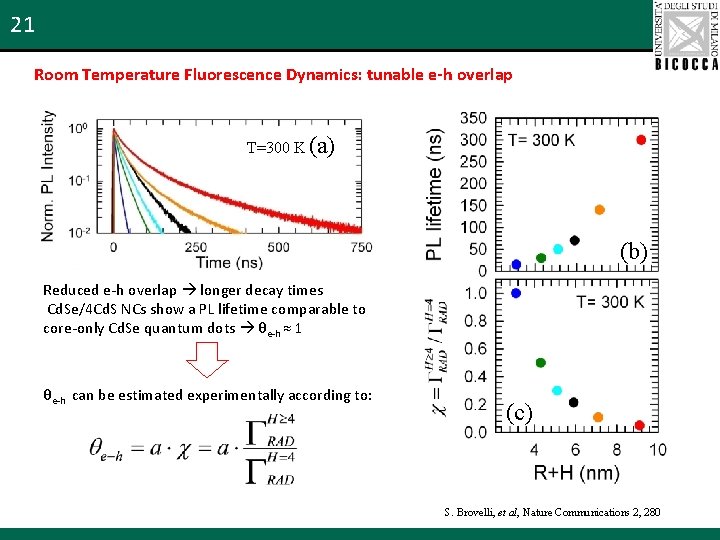
21 Room Temperature Fluorescence Dynamics: tunable e-h overlap T=300 K (a) (b) Reduced e-h overlap longer decay times Cd. Se/4 Cd. S NCs show a PL lifetime comparable to core-only Cd. Se quantum dots θe-h ≈ 1 θe-h can be estimated experimentally according to: (c) S. Brovelli, et al, Nature Communications 2, 280
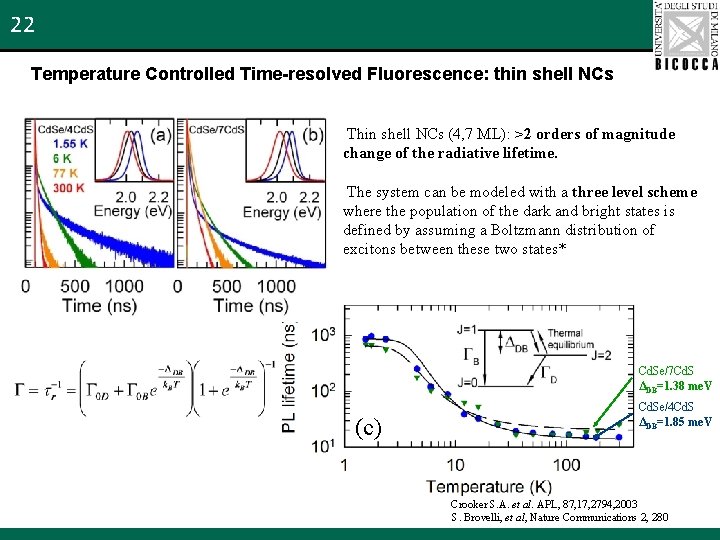
22 Temperature Controlled Time-resolved Fluorescence: thin shell NCs Thin shell NCs (4, 7 ML): >2 orders of magnitude change of the radiative lifetime. The system can be modeled with a three level scheme where the population of the dark and bright states is defined by assuming a Boltzmann distribution of excitons between these two states* Cd. Se/7 Cd. S ΔDB=1. 38 me. V (c) Cd. Se/4 Cd. S ΔDB=1. 85 me. V Crooker S. A. et al. APL, 87, 17, 2794, 2003 S. Brovelli, et al, Nature Communications 2, 280
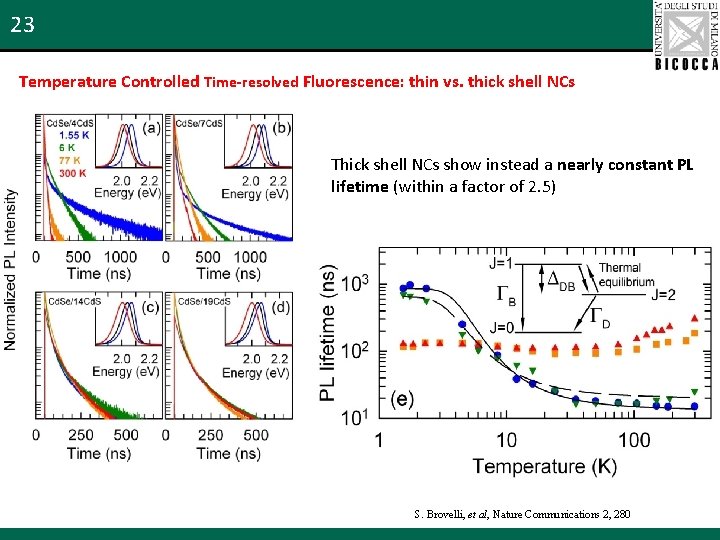
23 Temperature Controlled Time-resolved Fluorescence: thin vs. thick shell NCs Thick shell NCs show instead a nearly constant PL lifetime (within a factor of 2. 5) S. Brovelli, et al, Nature Communications 2, 280
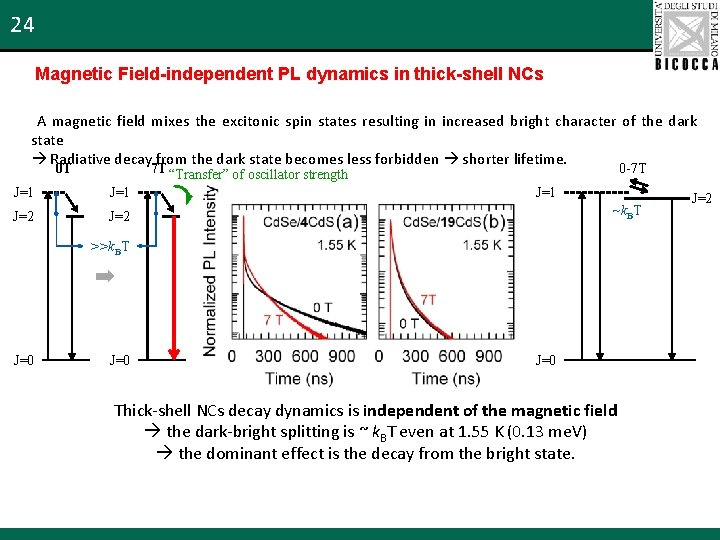
24 Magnetic Field-independent PL dynamics in thick-shell NCs A magnetic field mixes the excitonic spin states resulting in increased bright character of the dark state Radiative decay from the dark state becomes less forbidden shorter lifetime. 0 T J=1 J=2 0 -7 T 7 T “Transfer” of oscillator strength J=1 ~k. BT J=2 >>k. BT J=0 J=0 Thick-shell NCs decay dynamics is independent of the magnetic field the dark-bright splitting is ~ k. BT even at 1. 55 K (0. 13 me. V) the dominant effect is the decay from the bright state. J=2
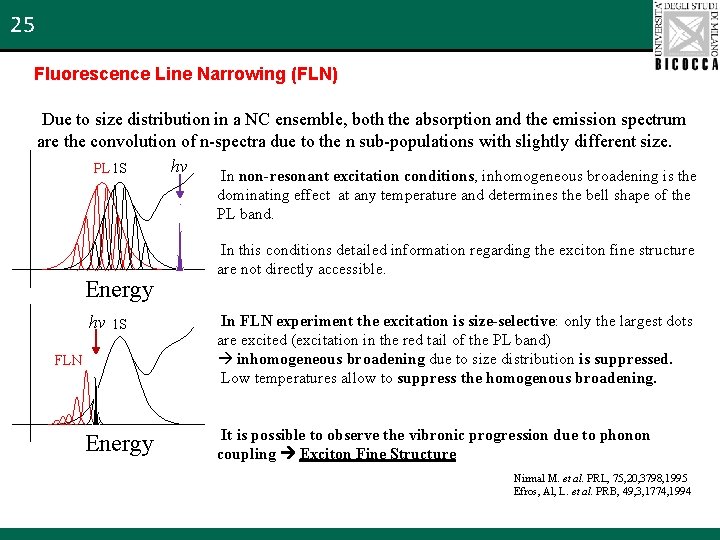
25 Fluorescence Line Narrowing (FLN) Due to size distribution in a NC ensemble, both the absorption and the emission spectrum are the convolution of n-spectra due to the n sub-populations with slightly different size. hν PL 1 S In non-resonant excitation conditions, inhomogeneous broadening is the dominating effect at any temperature and determines the bell shape of the PL band. Energy hν 1 S FLN Energy In this conditions detailed information regarding the exciton fine structure are not directly accessible. In FLN experiment the excitation is size-selective: only the largest dots are excited (excitation in the red tail of the PL band) inhomogeneous broadening due to size distribution is suppressed. Low temperatures allow to suppress the homogenous broadening. It is possible to observe the vibronic progression due to phonon coupling Exciton Fine Structure Nirmal M. et al. PRL, 75, 20, 3798, 1995 Efros, Al, L. et al. PRB, 49, 3, 1774, 1994
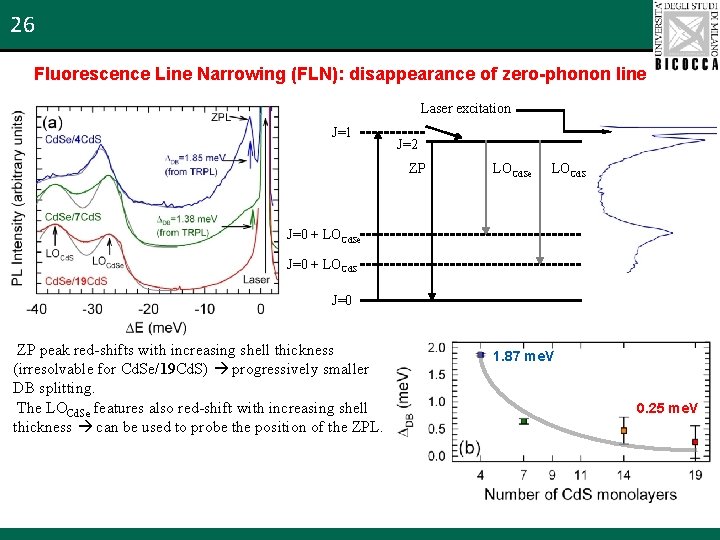
26 Fluorescence Line Narrowing (FLN): disappearance of zero-phonon line Laser excitation J=1 J=2 ZP LOCd. Se LOCd. S J=0 + LOCd. Se J=0 + LOCd. S J=0 ZP peak red-shifts with increasing shell thickness (irresolvable for Cd. Se/19 Cd. S) progressively smaller DB splitting. The LOCd. Se features also red-shift with increasing shell thickness can be used to probe the position of the ZPL. 1. 87 me. V 0. 25 me. V
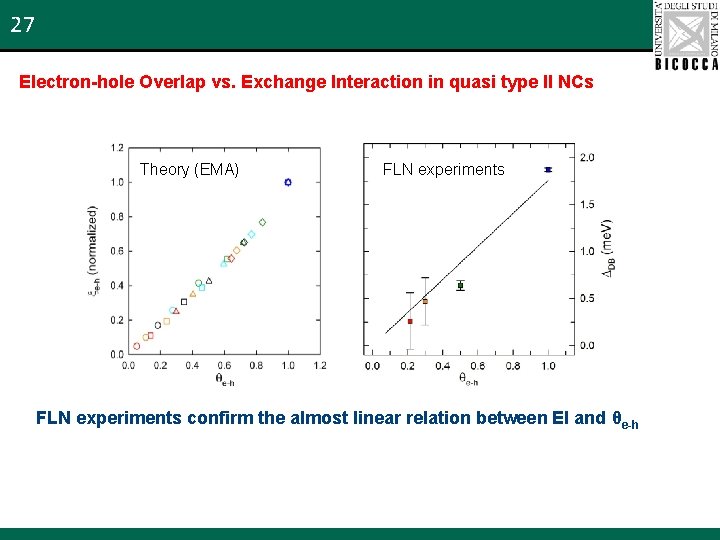
27 Electron-hole Overlap vs. Exchange Interaction in quasi type II NCs Theory (EMA) FLN experiments confirm the almost linear relation between EI and θe-h
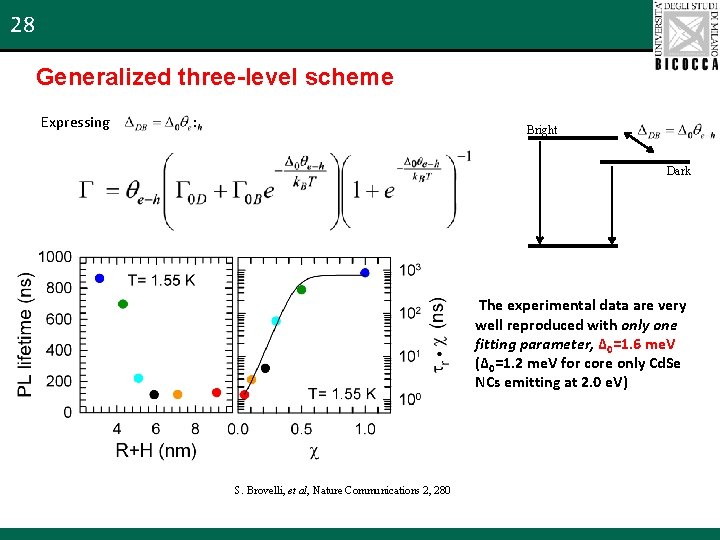
28 Generalized three-level scheme Expressing : Bright Dark The experimental data are very well reproduced with only one fitting parameter, Δ 0=1. 6 me. V (Δ 0=1. 2 me. V for core only Cd. Se NCs emitting at 2. 0 e. V) S. Brovelli, et al, Nature Communications 2, 280
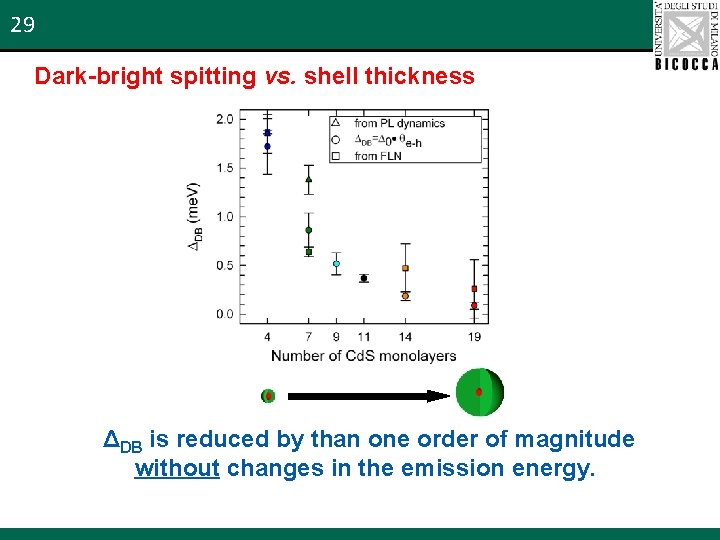
29 Dark-bright spitting vs. shell thickness ΔDB is reduced by than one order of magnitude without changes in the emission energy.
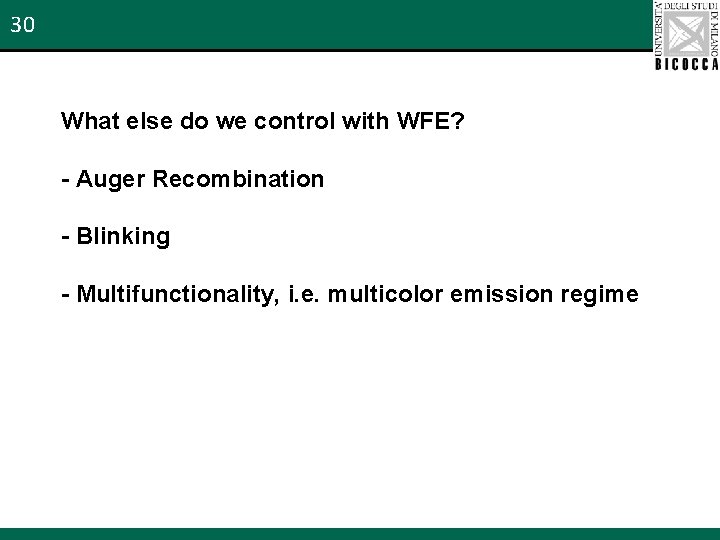
30 What else do we control with WFE? - Auger Recombination - Blinking - Multifunctionality, i. e. multicolor emission regime
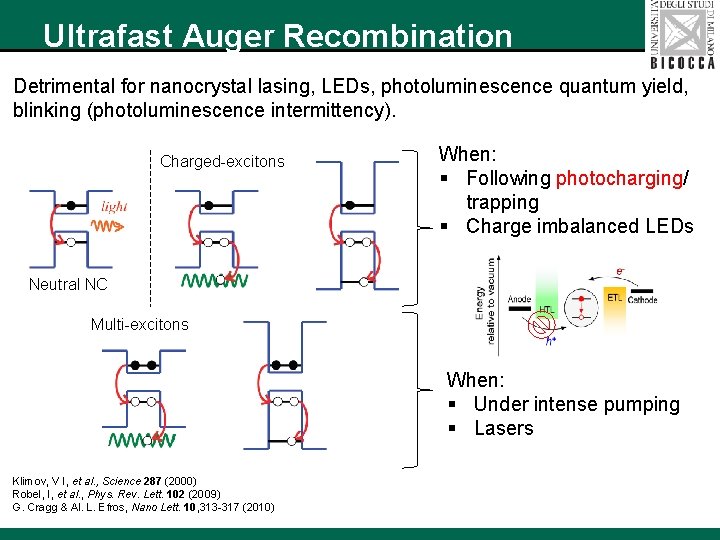
Ultrafast Auger Recombination Detrimental for nanocrystal lasing, LEDs, photoluminescence quantum yield, blinking (photoluminescence intermittency). Charged-excitons When: § Following photocharging/ trapping § Charge imbalanced LEDs Neutral NC Multi-excitons When: § Under intense pumping § Lasers Klimov, V I, et al. , Science 287 (2000) Robel, I, et al. , Phys. Rev. Lett. 102 (2009) G. Cragg & Al. L. Efros, Nano Lett. 10, 313 -317 (2010)
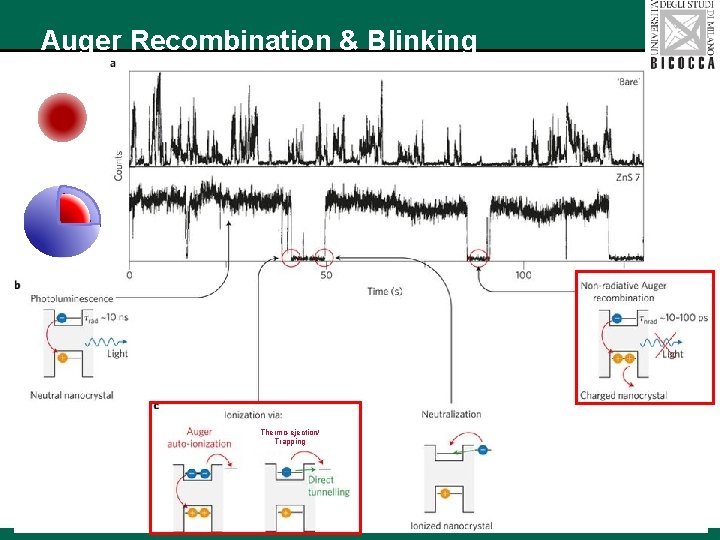
Auger Recombination & Blinking Thermo-ejection/ Trapping
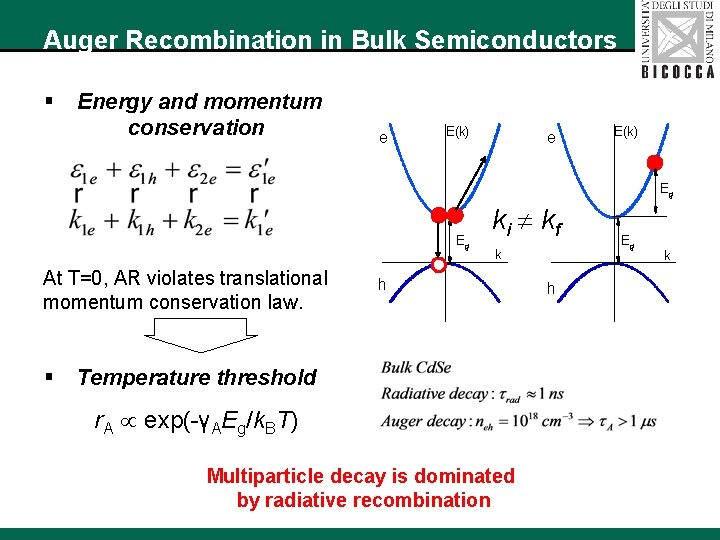
Auger Recombination in Bulk Semiconductors § Energy and momentum conservation e E(k) Eg Eg At T=0, AR violates translational momentum conservation law. § ki kf k h Temperature threshold r. A exp(-γAEg/k. BT) Multiparticle decay is dominated by radiative recombination h Eg k
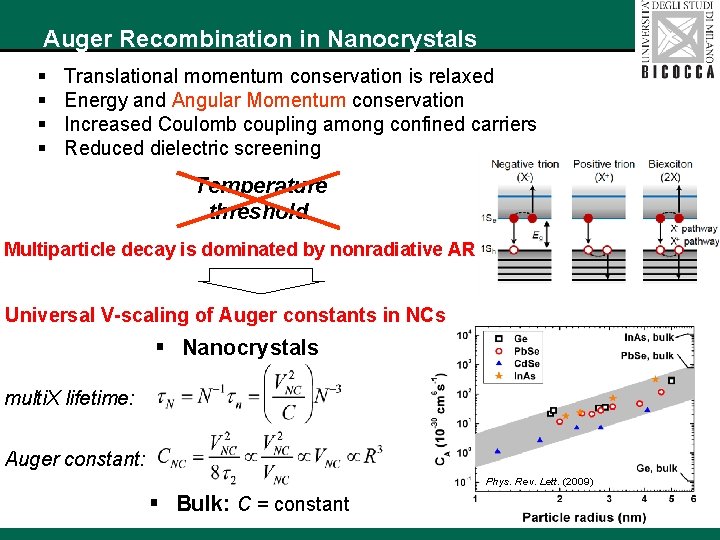
Auger Recombination in Nanocrystals § § Translational momentum conservation is relaxed Energy and Angular Momentum conservation Increased Coulomb coupling among confined carriers Reduced dielectric screening Temperature threshold Multiparticle decay is dominated by nonradiative AR Universal V-scaling of Auger constants in NCs § Nanocrystals multi. X lifetime: Auger constant: Phys. Rev. Lett. (2009) § Bulk: C = constant
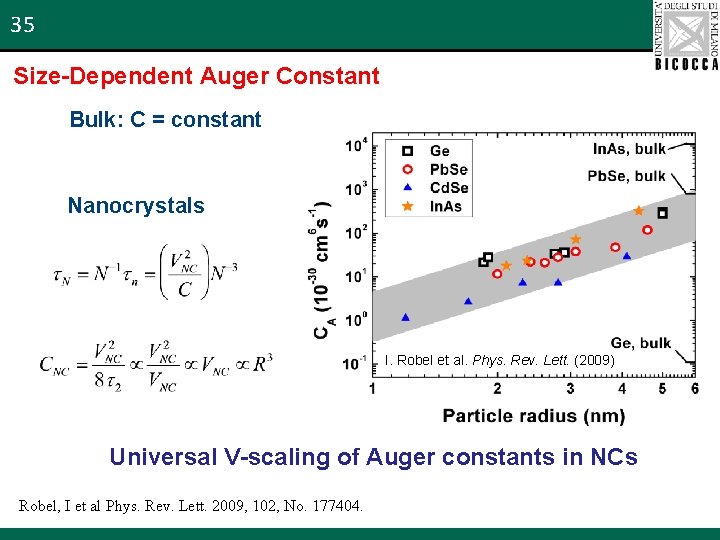
35 Size-Dependent Auger Constant Bulk: C = constant Nanocrystals I. Robel et al. Phys. Rev. Lett. (2009) Universal V-scaling of Auger constants in NCs Robel, I et al Phys. Rev. Lett. 2009, 102, No. 177404.
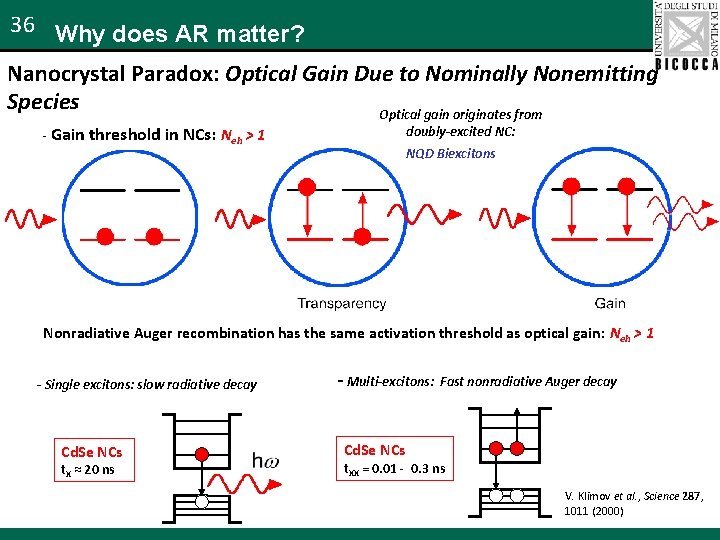
36 Why does AR matter? Nanocrystal Paradox: Optical Gain Due to Nominally Nonemitting Species Optical gain originates from doubly-excited NC: - Gain threshold in NCs: Neh > 1 NQD Biexcitons Nonradiative Auger recombination has the same activation threshold as optical gain: Neh > 1 - Single excitons: slow radiative decay Cd. Se NCs t. X ≈ 20 ns - Multi-excitons: Fast nonradiative Auger decay Cd. Se NCs t. XX = 0. 01 - 0. 3 ns V. Klimov et al. , Science 287, 1011 (2000)
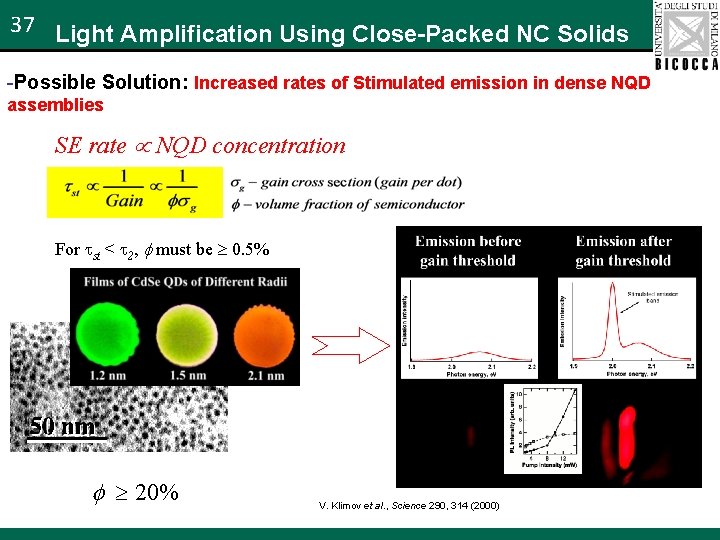
37 Light Amplification Using Close-Packed NC Solids -Possible Solution: Increased rates of Stimulated emission in dense NQD assemblies SE rate NQD concentration For st < 2, must be 0. 5% 20% V. Klimov et al. , Science 290, 314 (2000)
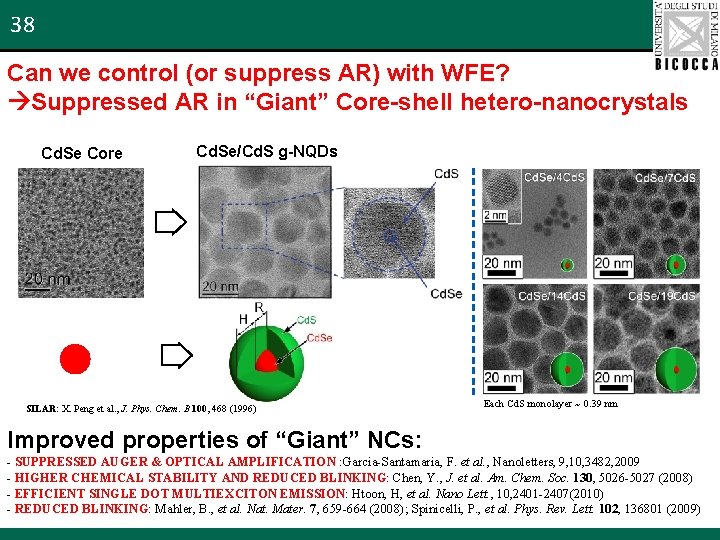
38 Can we control (or suppress AR) with WFE? Suppressed AR in “Giant” Core-shell hetero-nanocrystals Cd. Se Core Cd. Se/Cd. S g-NQDs SILAR: X. Peng et al. , J. Phys. Chem. B 100, 468 (1996) Each Cd. S monolayer ~ 0. 39 nm Improved properties of “Giant” NCs: - SUPPRESSED AUGER & OPTICAL AMPLIFICATION : Garcia-Santamaria, F. et al. , Nanoletters, 9, 10, 3482, 2009 - HIGHER CHEMICAL STABILITY AND REDUCED BLINKING: Chen, Y. , J. et al. Am. Chem. Soc. 130, 5026 -5027 (2008) - EFFICIENT SINGLE DOT MULTIEXCITON EMISSION: Htoon, H, et al. Nano Lett. , 10, 2401 -2407(2010) - REDUCED BLINKING: Mahler, B. , et al. Nat. Mater. 7, 659 -664 (2008); Spinicelli, P. , et al. Phys. Rev. Lett. 102, 136801 (2009)
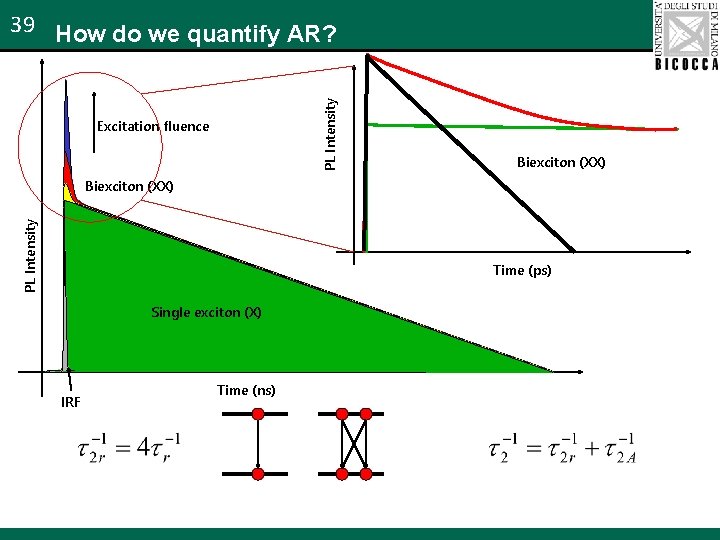
PL Intensity 39 How do we quantify AR? Excitation fluence Biexciton (XX) PL Intensity Biexciton (XX) Time (ps) Single exciton (X) IRF Time (ns)
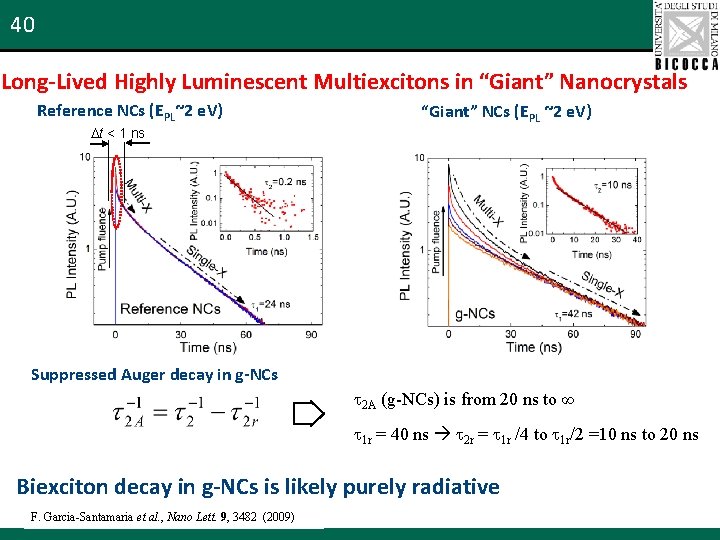
40 Long-Lived Highly Luminescent Multiexcitons in “Giant” Nanocrystals Reference NCs (EPL~2 e. V) t < 1 ns “Giant” NCs (EPL ~2 e. V) Suppressed Auger decay in g-NCs 2 A (g-NCs) is from 20 ns to ∞ 1 r = 40 ns 2 r = 1 r /4 to 1 r/2 =10 ns to 20 ns Biexciton decay in g-NCs is likely purely radiative F. Garcia-Santamaria et al. , Nano Lett. 9, 3482 (2009)
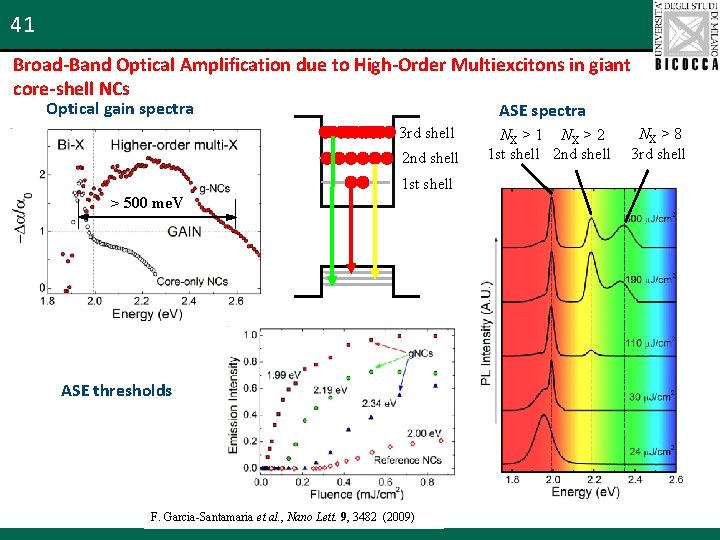
41 Broad-Band Optical Amplification due to High-Order Multiexcitons in giant core-shell NCs Optical gain spectra ASE spectra 3 rd shell 2 nd shell 1 st shell > 500 me. V ASE thresholds F. Garcia-Santamaria et al. , Nano Lett. 9, 3482 (2009) NX > 1 NX > 2 1 st shell 2 nd shell NX > 8 3 rd shell
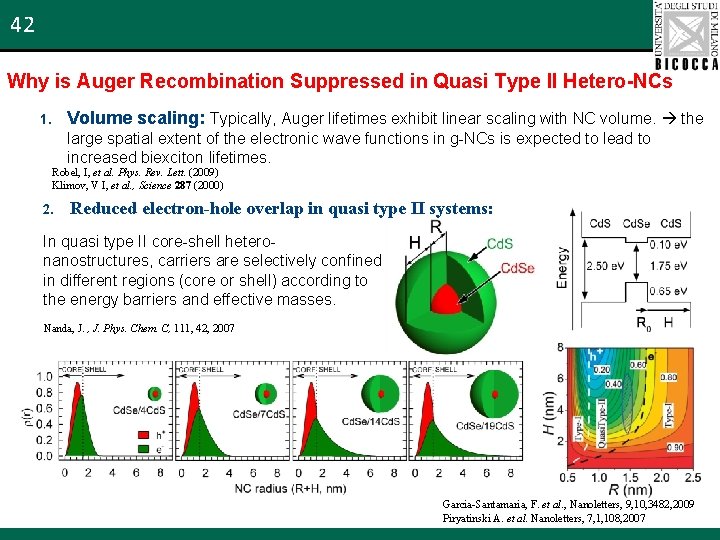
42 Why is Auger Recombination Suppressed in Quasi Type II Hetero-NCs Volume scaling: Typically, Auger lifetimes exhibit linear scaling with NC volume. the 1. large spatial extent of the electronic wave functions in g-NCs is expected to lead to increased biexciton lifetimes. Robel, I, et al. Phys. Rev. Lett. (2009) Klimov, V I, et al. , Science 287 (2000) 2. Reduced electron-hole overlap in quasi type II systems: In quasi type II core-shell heteronanostructures, carriers are selectively confined in different regions (core or shell) according to the energy barriers and effective masses. Nanda, J. Phys. Chem. C, 111, 42, 2007 Garcia-Santamaria, F. et al. , Nanoletters, 9, 10, 3482, 2009 Piryatinski A. et al. Nanoletters, 7, 1, 108, 2007
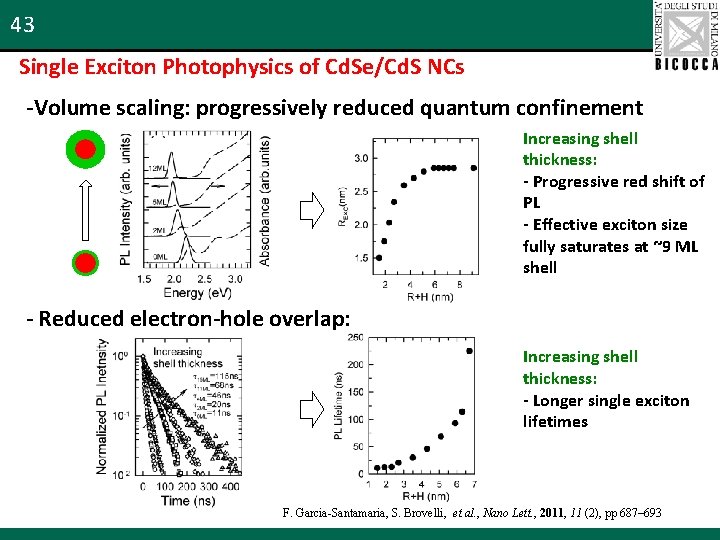
43 Single Exciton Photophysics of Cd. Se/Cd. S NCs -Volume scaling: progressively reduced quantum confinement Increasing shell thickness: - Progressive red shift of PL - Effective exciton size fully saturates at ~9 ML shell - Reduced electron-hole overlap: Increasing shell thickness: - Longer single exciton lifetimes F. Garcia-Santamaria, S. Brovelli, et al. , Nano Lett. , 2011, 11 (2), pp 687– 693
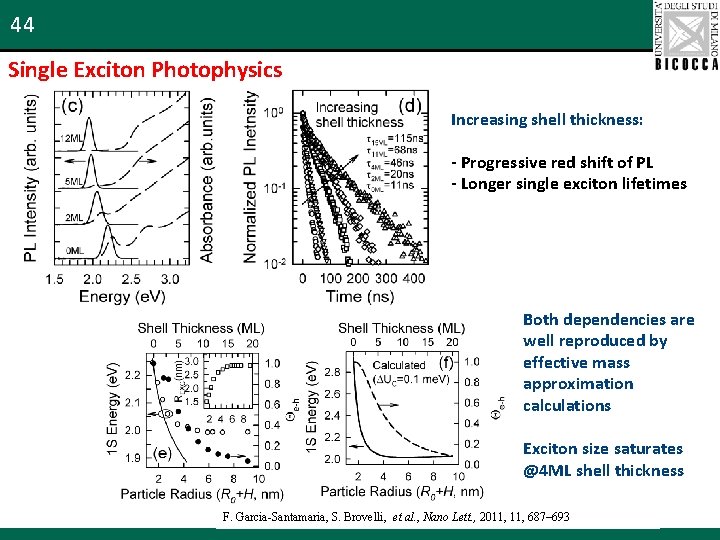
44 Single Exciton Photophysics Increasing shell thickness: - Progressive red shift of PL - Longer single exciton lifetimes Both dependencies are well reproduced by effective mass approximation calculations Exciton size saturates @4 ML shell thickness F. Garcia-Santamaria, S. Brovelli, et al. , Nano Lett. , 2011, 687– 693
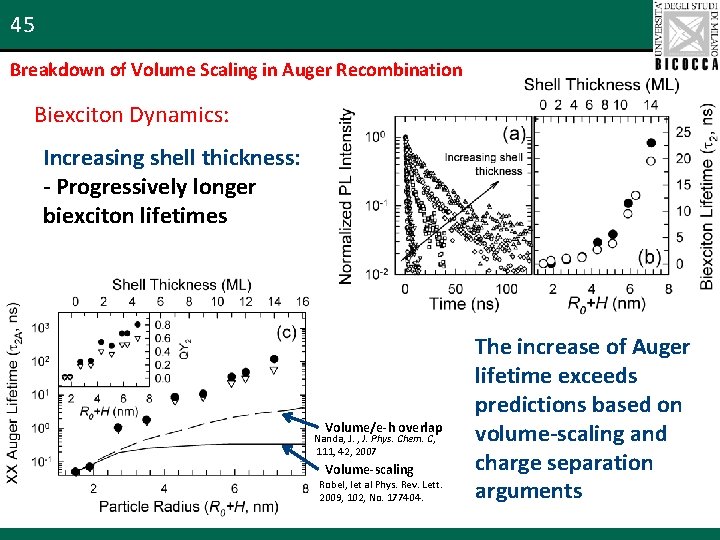
45 Breakdown of Volume Scaling in Auger Recombination Biexciton Dynamics: Increasing shell thickness: - Progressively longer biexciton lifetimes Volume/e-h overlap Nanda, J. Phys. Chem. C, 111, 42, 2007 Volume-scaling Robel, Iet al Phys. Rev. Lett. 2009, 102, No. 177404. The increase of Auger lifetime exceeds predictions based on volume-scaling and charge separation arguments
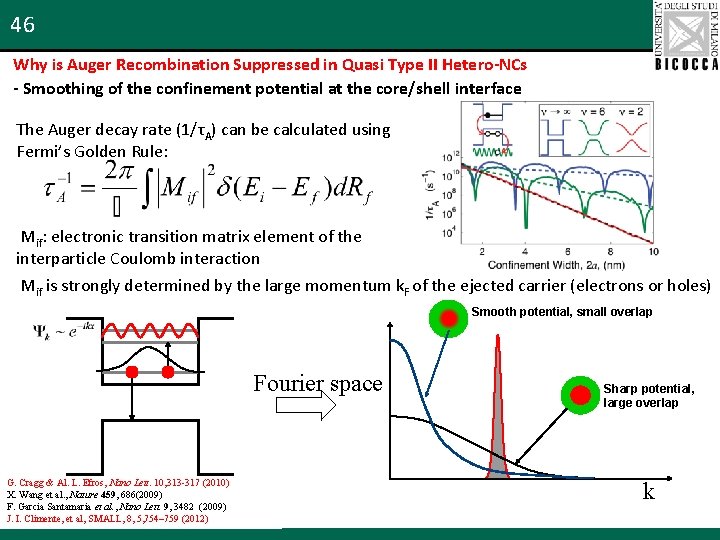
46 Why is Auger Recombination Suppressed in Quasi Type II Hetero-NCs - Smoothing of the confinement potential at the core/shell interface The Auger decay rate (1/τA) can be calculated using Fermi’s Golden Rule: Large k. F Mif: electronic transition matrix element of the interparticle Coulomb interaction Mif is strongly determined by the large momentum k. F of the ejected carrier (electrons or holes) Smooth potential, small overlap Fourier space G. Cragg & Al. L. Efros, Nano Lett. 10, 313 -317 (2010) X. Wang et al. , Nature 459, 686(2009) F. Garcia Santamaria et al. , Nano Lett. 9, 3482 (2009) J. I. Climente, et al, SMALL, 8, 5, 754– 759 (2012) Sharp potential, large overlap k
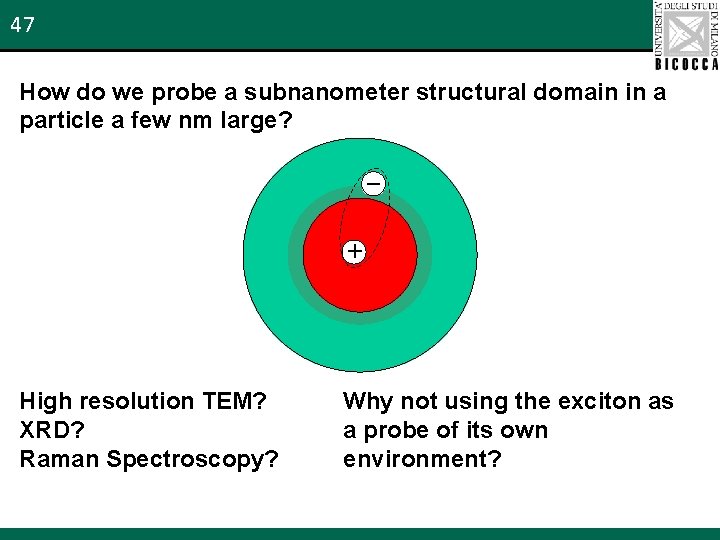
47 How do we probe a subnanometer structural domain in a particle a few nm large? _ + High resolution TEM? XRD? Raman Spectroscopy? Why not using the exciton as a probe of its own environment?
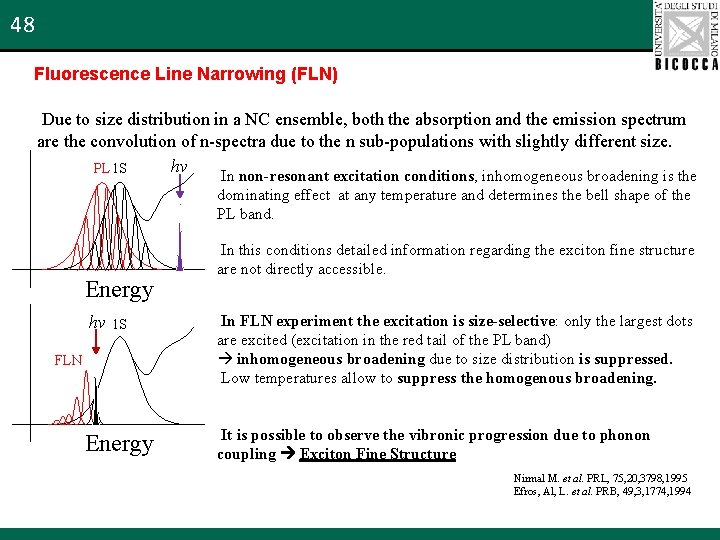
48 Fluorescence Line Narrowing (FLN) Due to size distribution in a NC ensemble, both the absorption and the emission spectrum are the convolution of n-spectra due to the n sub-populations with slightly different size. hν PL 1 S In non-resonant excitation conditions, inhomogeneous broadening is the dominating effect at any temperature and determines the bell shape of the PL band. Energy hν 1 S FLN Energy In this conditions detailed information regarding the exciton fine structure are not directly accessible. In FLN experiment the excitation is size-selective: only the largest dots are excited (excitation in the red tail of the PL band) inhomogeneous broadening due to size distribution is suppressed. Low temperatures allow to suppress the homogenous broadening. It is possible to observe the vibronic progression due to phonon coupling Exciton Fine Structure Nirmal M. et al. PRL, 75, 20, 3798, 1995 Efros, Al, L. et al. PRB, 49, 3, 1774, 1994
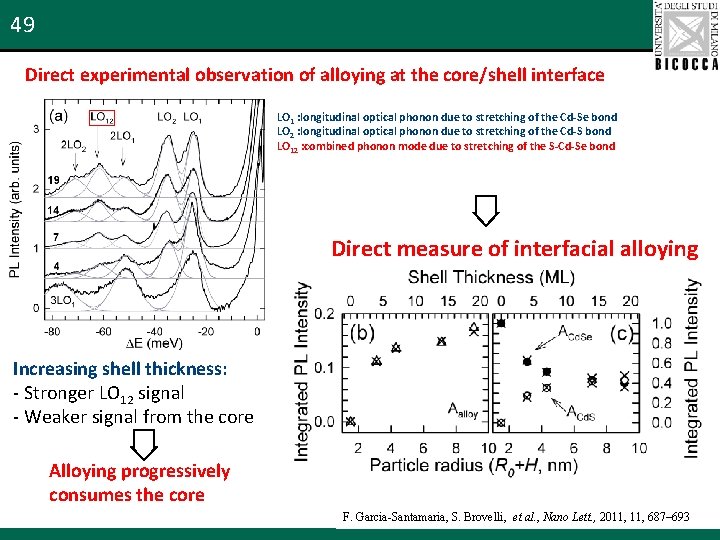
49 Direct experimental observation of alloying at the core/shell interface LO 1 : longitudinal optical phonon due to stretching of the Cd-Se bond LO 2 : longitudinal optical phonon due to stretching of the Cd-S bond LO 12 : combined phonon mode due to stretching of the S-Cd-Se bond Direct measure of interfacial alloying Increasing shell thickness: - Stronger LO 12 signal - Weaker signal from the core Alloying progressively consumes the core F. Garcia-Santamaria, S. Brovelli, et al. , Nano Lett. , 2011, 687– 693
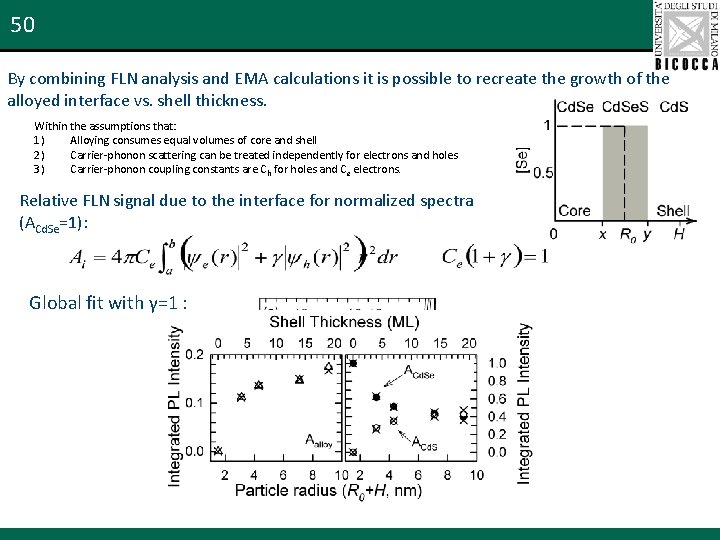
50 By combining FLN analysis and EMA calculations it is possible to recreate the growth of the alloyed interface vs. shell thickness. Within the assumptions that: 1) Alloying consumes equal volumes of core and shell 2) Carrier-phonon scattering can be treated independently for electrons and holes 3) Carrier-phonon coupling constants are Ch for holes and Ce electrons. Relative FLN signal due to the interface for normalized spectra (ACd. Se=1): Global fit with γ=1 :
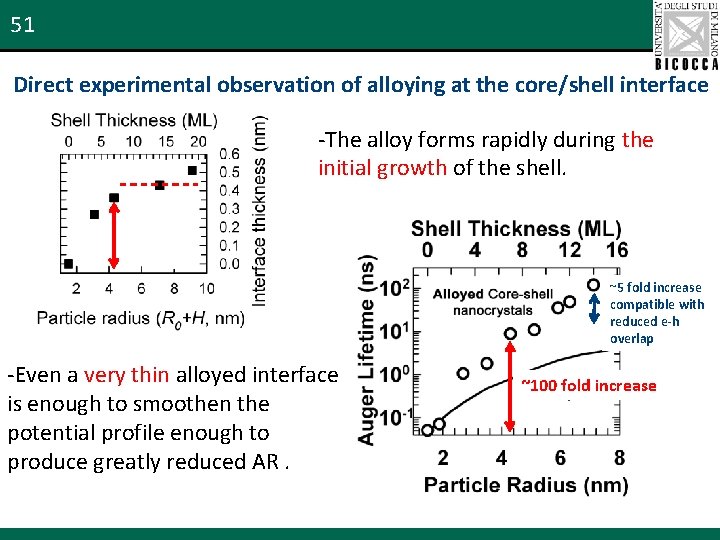
51 Direct experimental observation of alloying at the core/shell interface -The alloy forms rapidly during the initial growth of the shell. ~5 fold increase compatible with reduced e-h overlap -Even a very thin alloyed interface is enough to smoothen the potential profile enough to produce greatly reduced AR. ~100 fold increase
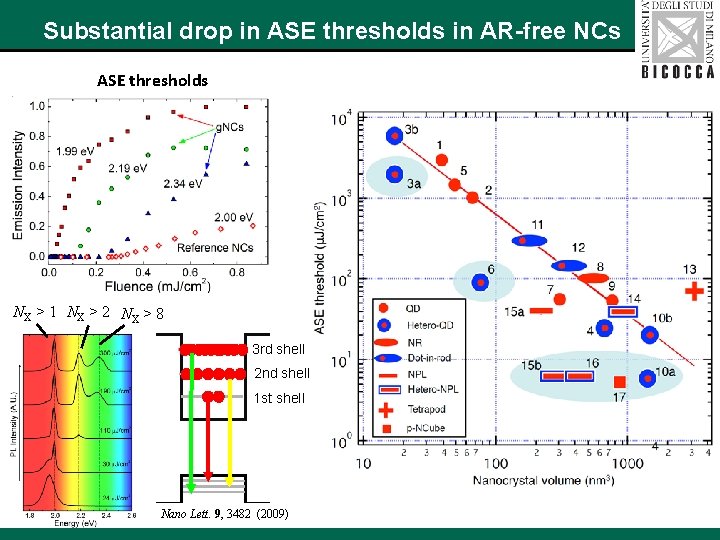
Substantial drop in ASE thresholds in AR-free NCs ASE thresholds NX > 1 NX > 2 NX > 8 3 rd shell 2 nd shell 1 st shell Nano Lett. 9, 3482 (2009)
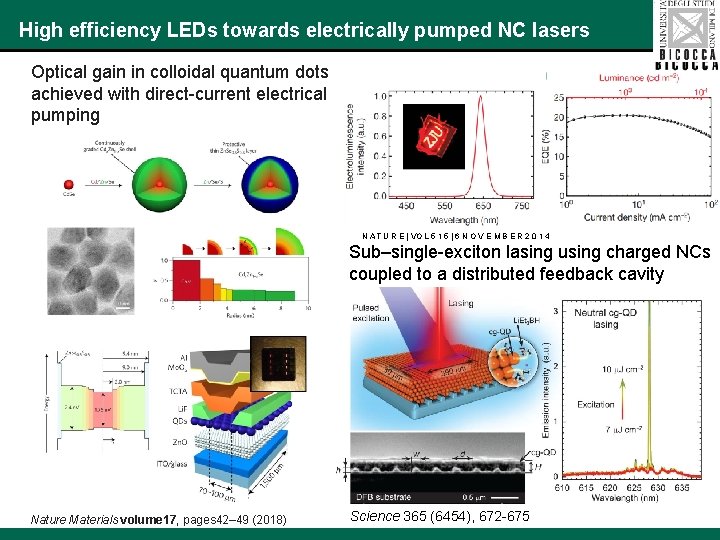
High efficiency LEDs towards electrically pumped NC lasers Optical gain in colloidal quantum dots achieved with direct-current electrical pumping N AT U R E | VO L 5 1 5 | 6 N O V E M B E R 2 0 1 4 Sub–single-exciton lasing using charged NCs coupled to a distributed feedback cavity Nature Materials volume 17, pages 42– 49 (2018) Science 365 (6454), 672 -675
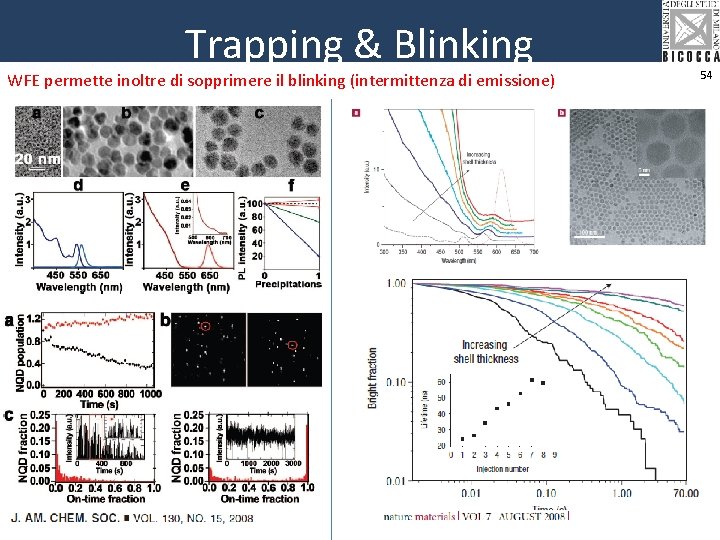
Trapping & Blinking WFE permette inoltre di sopprimere il blinking (intermittenza di emissione) 54
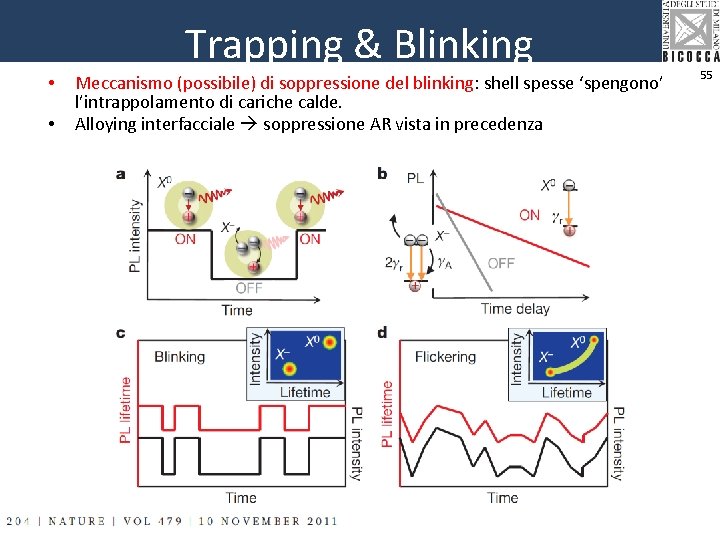
Trapping & Blinking • • Meccanismo (possibile) di soppressione del blinking: shell spesse ‘spengono’ l’intrappolamento di cariche calde. Alloying interfacciale soppressione AR vista in precedenza 55
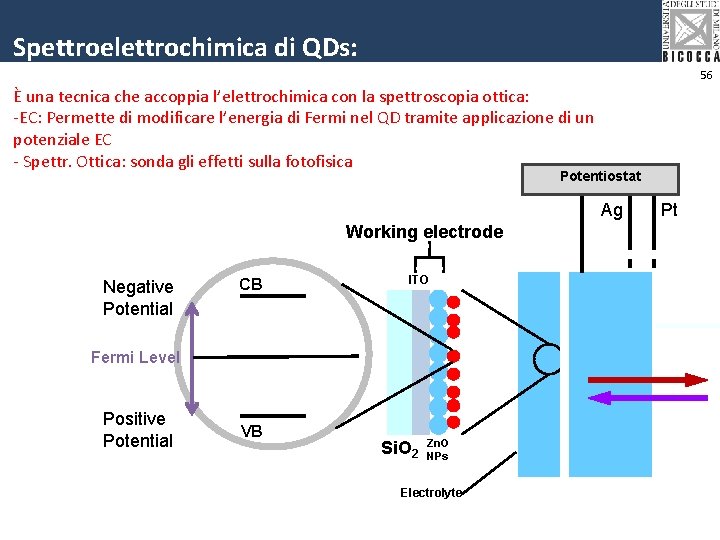
Spettroelettrochimica di QDs: 56 È una tecnica che accoppia l’elettrochimica con la spettroscopia ottica: -EC: Permette di modificare l’energia di Fermi nel QD tramite applicazione di un potenziale EC - Spettr. Ottica: sonda gli effetti sulla fotofisica Potentiostat Ag Working electrode Negative Potential CB ITO Fermi Level Positive Potential VB Si. O 2 Zn. O NPs Electrolyte Pt
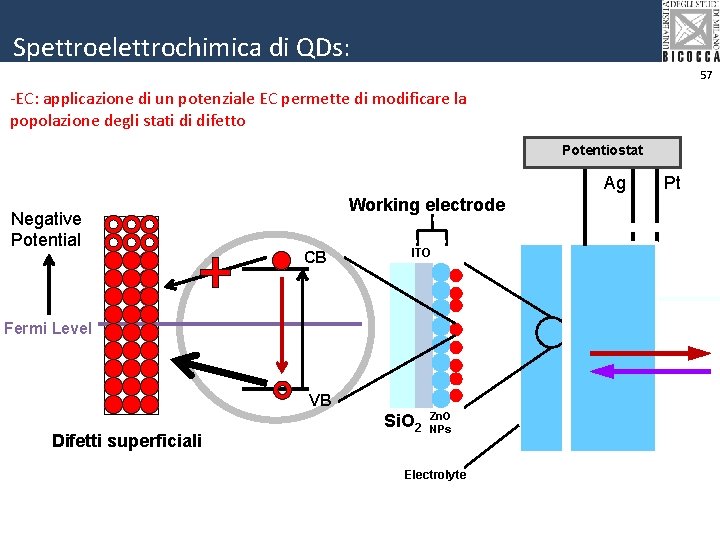
Spettroelettrochimica di QDs: 57 -EC: applicazione di un potenziale EC permette di modificare la popolazione degli stati di difetto Potentiostat Ag Negative Potential Working electrode CB ITO Fermi Level VB Difetti superficiali Si. O 2 Zn. O NPs Electrolyte Pt
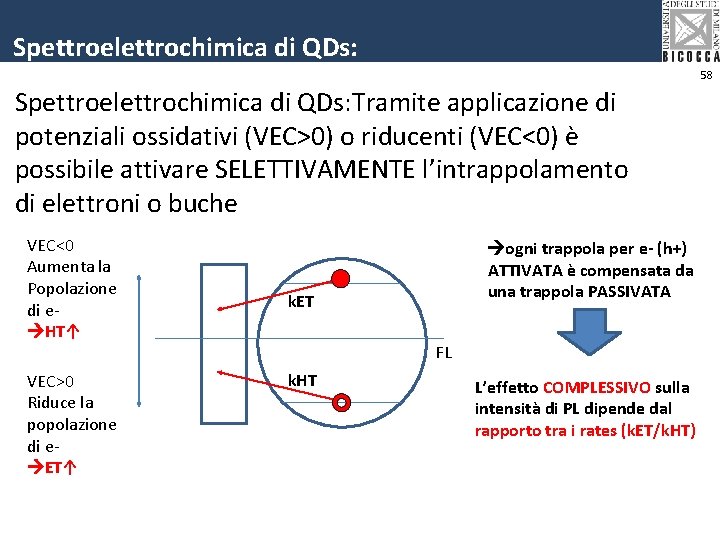
Spettroelettrochimica di QDs: 58 Spettroelettrochimica di QDs: Tramite applicazione di potenziali ossidativi (VEC>0) o riducenti (VEC<0) è possibile attivare SELETTIVAMENTE l’intrappolamento di elettroni o buche VEC<0 Aumenta la Popolazione di e HT↑ ogni trappola per e- (h+) ATTIVATA è compensata da una trappola PASSIVATA k. ET FL VEC>0 Riduce la popolazione di e ET↑ k. HT L’effetto COMPLESSIVO sulla intensità di PL dipende dal rapporto tra i rates (k. ET/k. HT)
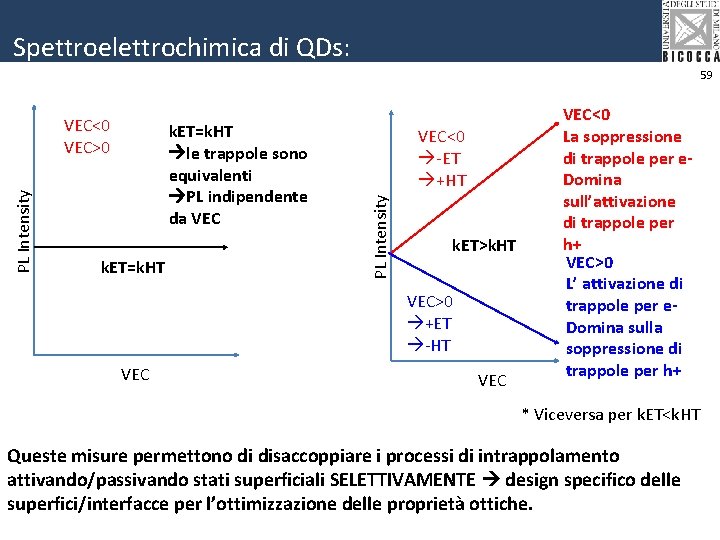
Spettroelettrochimica di QDs: 59 k. ET=k. HT le trappole sono equivalenti PL indipendente da VEC k. ET=k. HT VEC<0 -ET +HT PL Intensity VEC<0 VEC>0 k. ET>k. HT VEC>0 +ET -HT VEC VEC<0 La soppressione di trappole per e. Domina sull’attivazione di trappole per h+ VEC>0 L’ attivazione di trappole per e. Domina sulla soppressione di trappole per h+ * Viceversa per k. ET<k. HT Queste misure permettono di disaccoppiare i processi di intrappolamento attivando/passivando stati superficiali SELETTIVAMENTE design specifico delle superfici/interfacce per l’ottimizzazione delle proprietà ottiche.
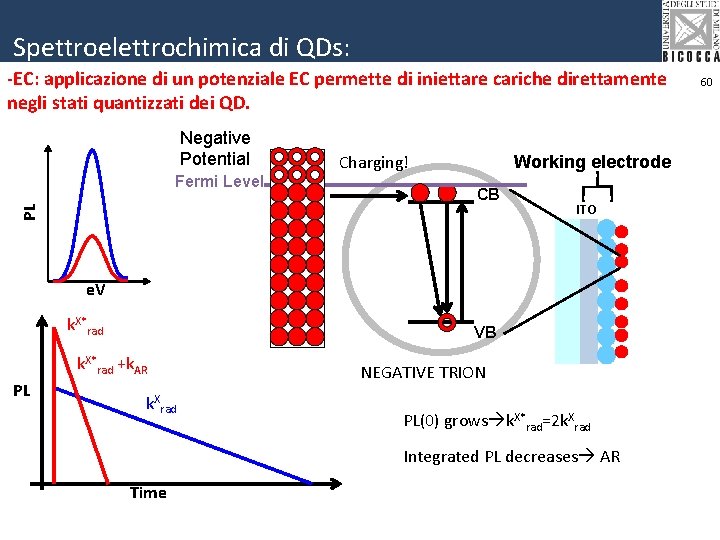
Spettroelettrochimica di QDs: -EC: applicazione di un potenziale EC permette di iniettare cariche direttamente negli stati quantizzati dei QD. Negative Potential Working electrode CB PL Fermi Level Charging! ITO e. V k. X*rad VB k. X*rad +k. AR PL k. Xrad NEGATIVE TRION PL(0) grows k. X*rad=2 k. Xrad Integrated PL decreases AR Time 60
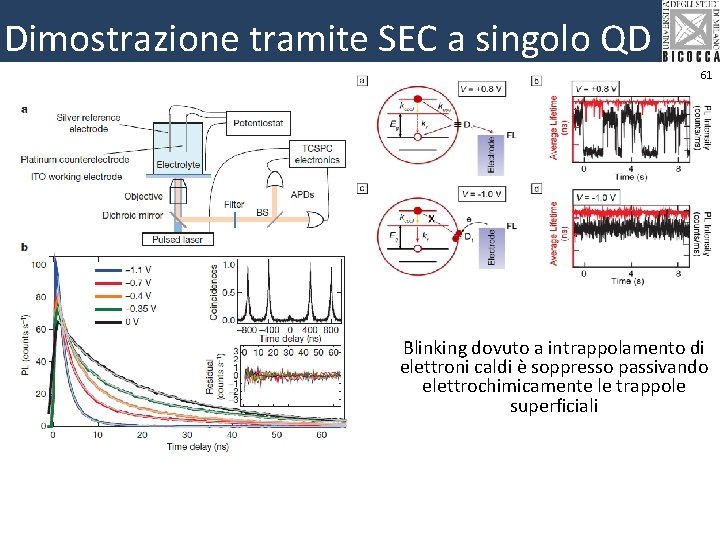
Dimostrazione tramite SEC a singolo QD 61 Blinking dovuto a intrappolamento di elettroni caldi è soppresso passivando elettrochimicamente le trappole superficiali
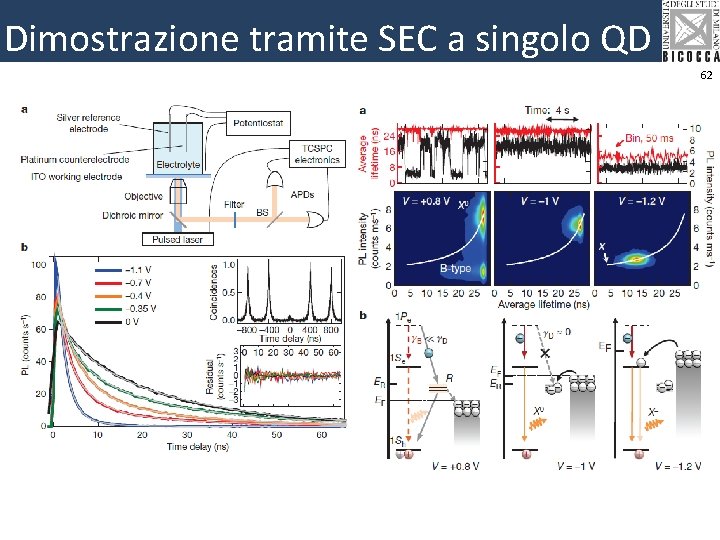
Dimostrazione tramite SEC a singolo QD 62
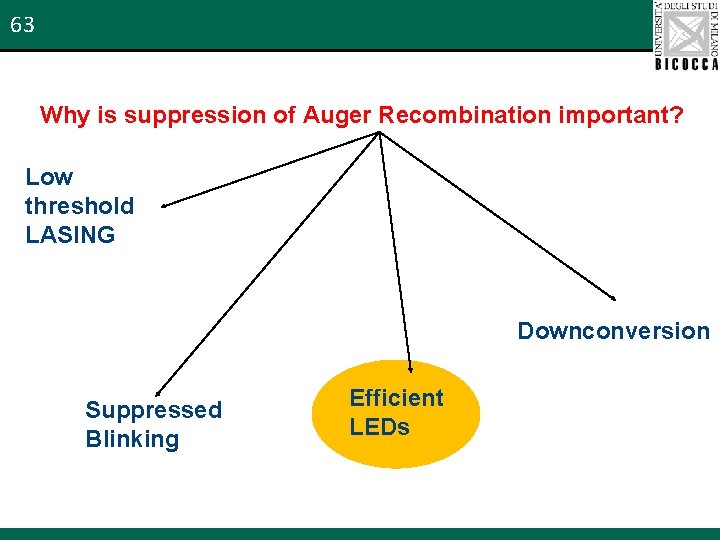
63 Why is suppression of Auger Recombination important? Low threshold LASING Downconversion Suppressed Blinking Efficient LEDs
History of quantum dots
History of quantum dots
Advanced semiconductor fundamentals
Advanced semiconductor fundamentals
Quantum physics vs mechanics
Quantum physics vs quantum mechanics
Colloid is a heterogeneous system
Difference between colloidal and crystalline precipitate
Mechanical entrapment coprecipitation
Classification of matter
Colloid examples
Collidal state
Difference between colloidal and crystalline precipitate
Types of gypsum products
Types of colloids and examples
Examples of colloids food
Colloidal silica gun mix
Occlusion in gravimetric analysis
Stability of sols
Stan jones blue
Protective colloids
Dots tb
What is the maximum number of pieces
Tbnow
Ca lewis dot structure
Mcl ucl
Roy lichtenstein pop art portraits
Think dots
Luka terhiris disebabkan oleh
Lesson 31 connect the dots lewis
Malaria means bad air
Dots tbc
Valence bond picture
Diplin dots
Schuffner dots malaria
99 dots
Ben day dots stencil
What do the dots represent
Connect 1 2 3 without crossing lines
Dot and screed plastering
Think dots differentiated instruction
Shilentnobyte
Ben-day dots
Helados dippin dots
Which artist utilized benday dots in his artwork
Black dots
Clear the blue dots
Trantas dots
Think dots differentiated instruction
Letter j in morse code
Tire rotation
Malaria parasite in thick film
Dots expansion
Exploding dots
Dot wall
Uppställning multiplikation
Delegerande ledarskap
Underlag för särskild löneskatt på pensionskostnader
Toppslätskivling effekt
Redogör för vad psykologi är
Borra hål för knoppar
Bris för vuxna
Mat för unga idrottare
Offentlig förvaltning