AN INTRODUCTION TO TRANSITION METAL CHEMISTRY INCREASING ENERGY
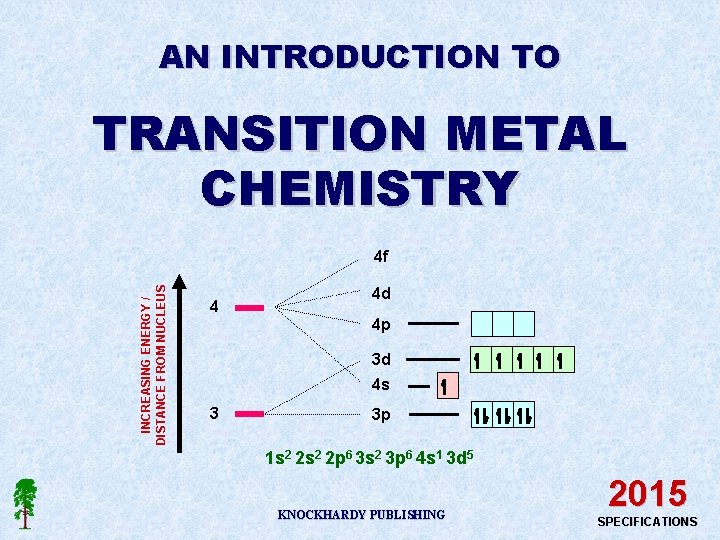
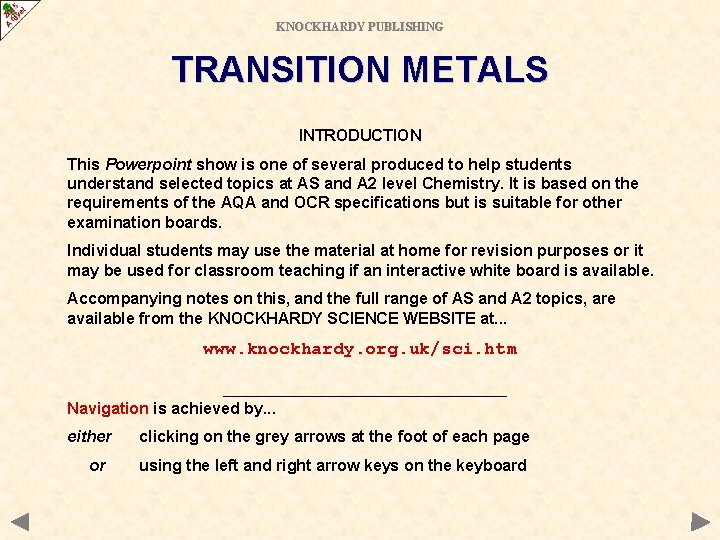
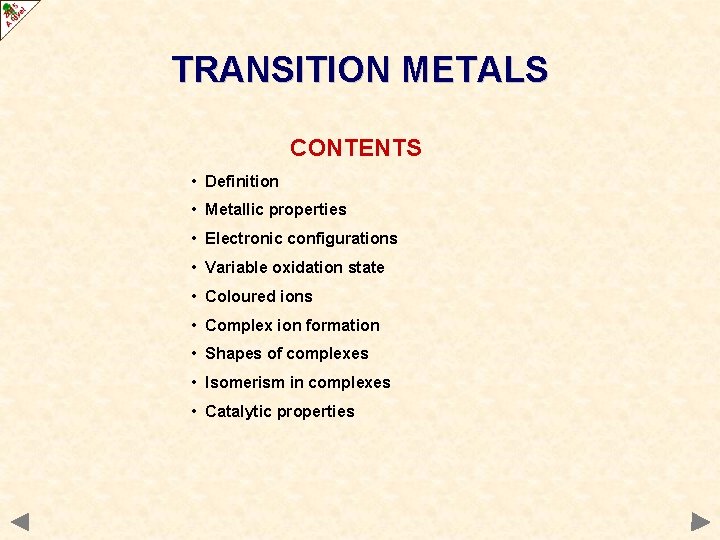
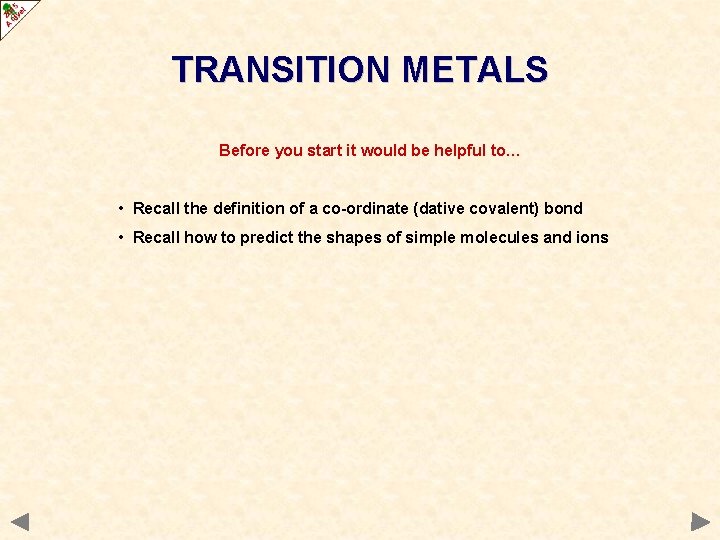
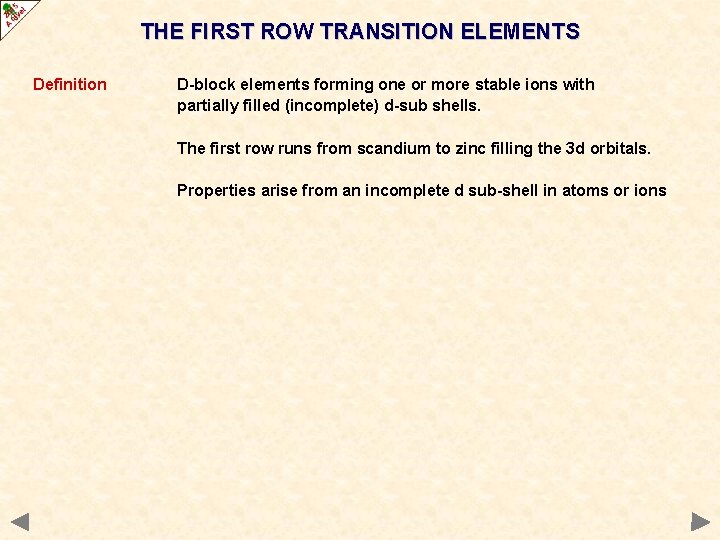
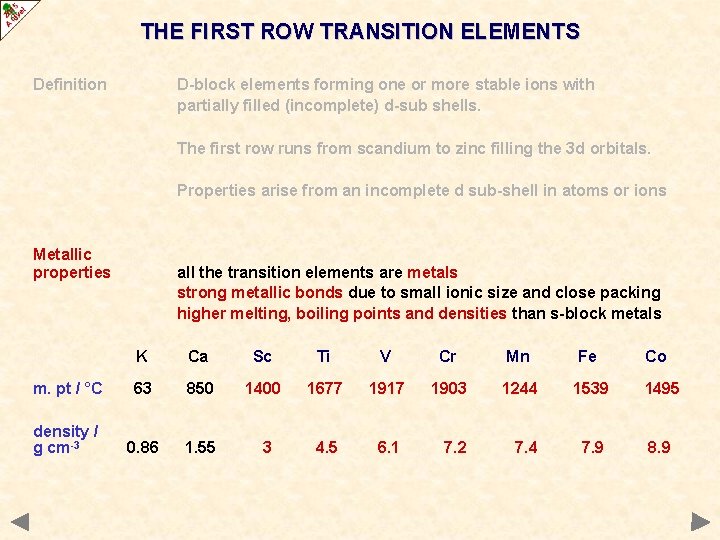
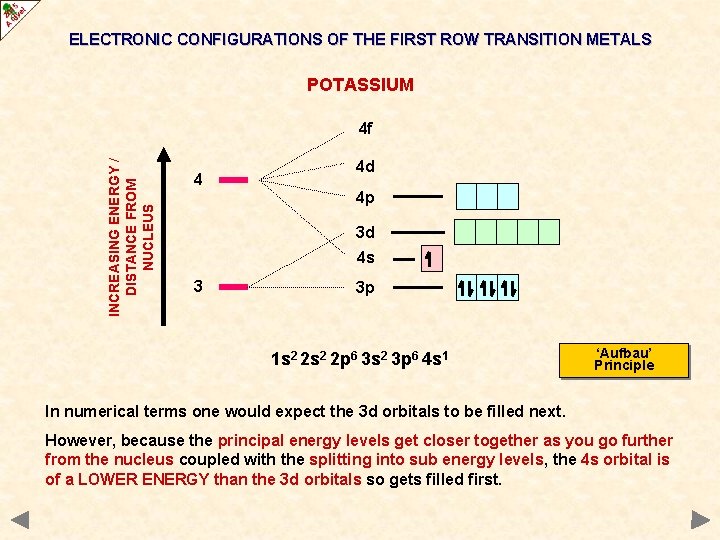
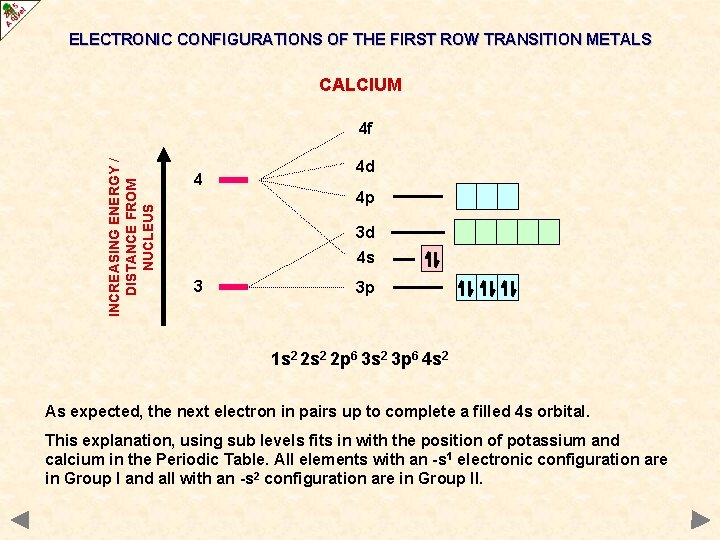
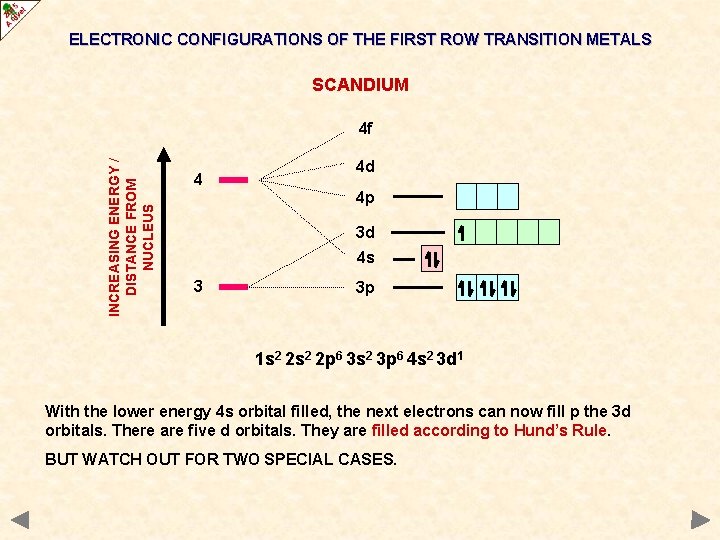
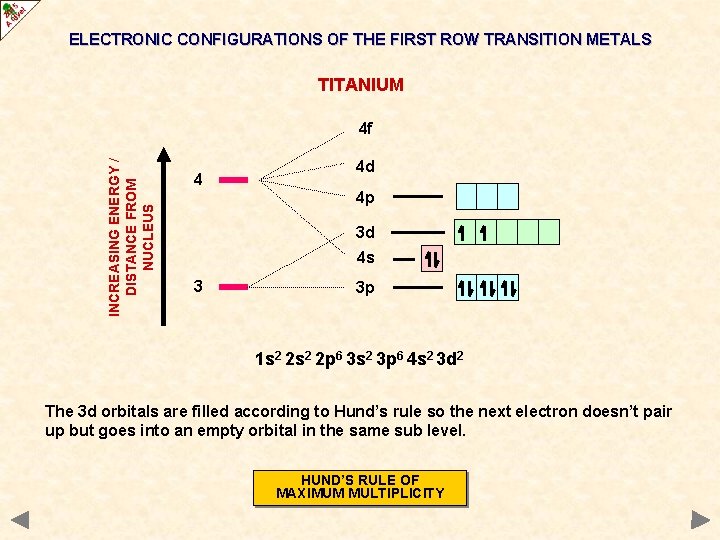
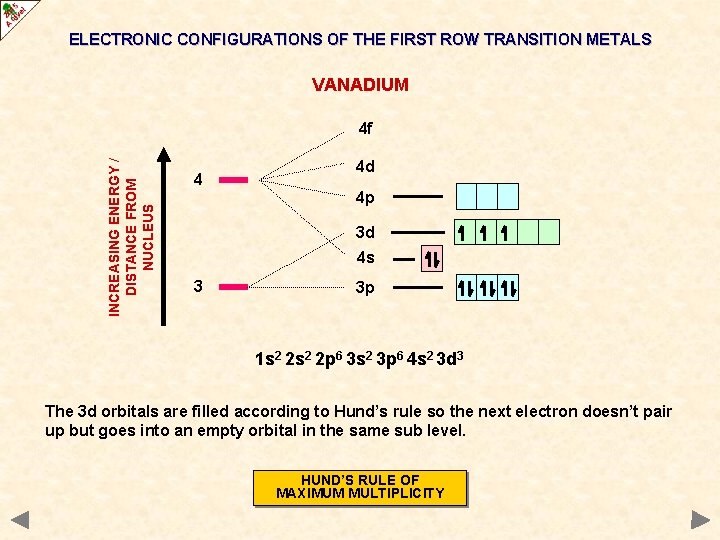
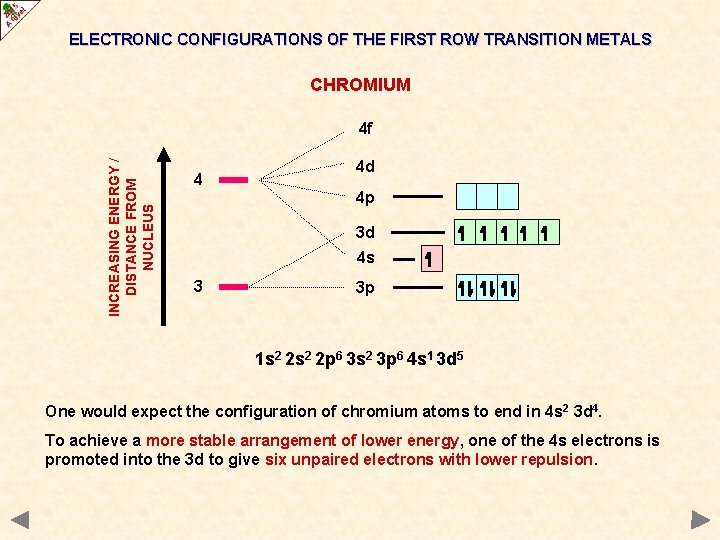
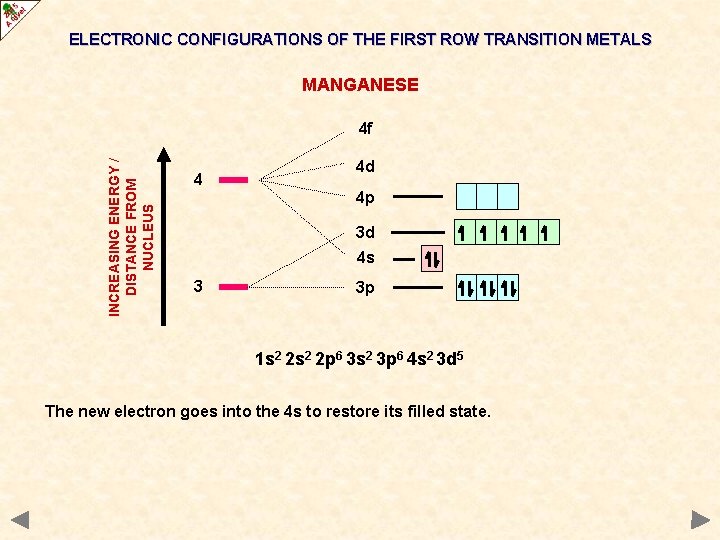
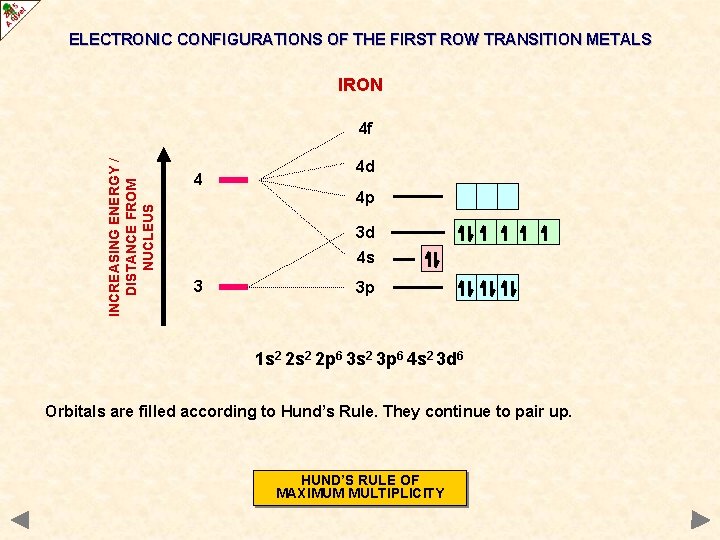
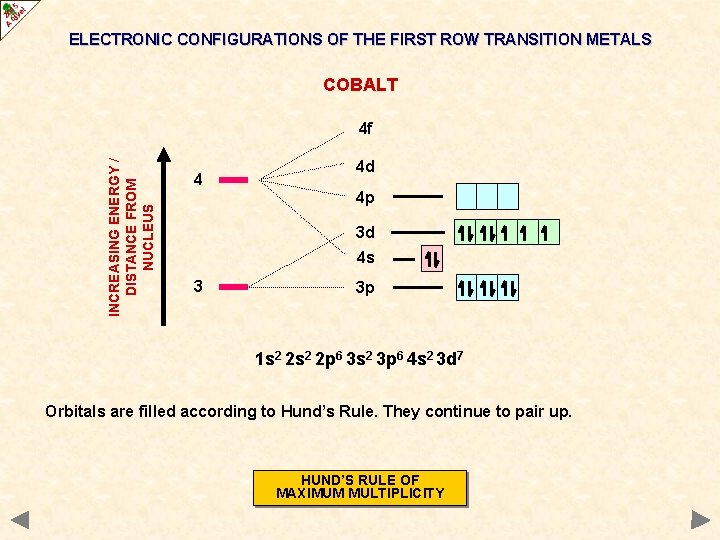
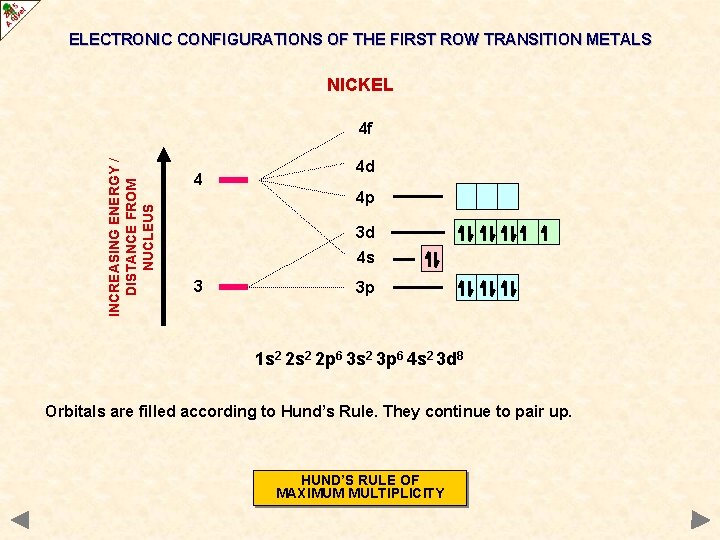
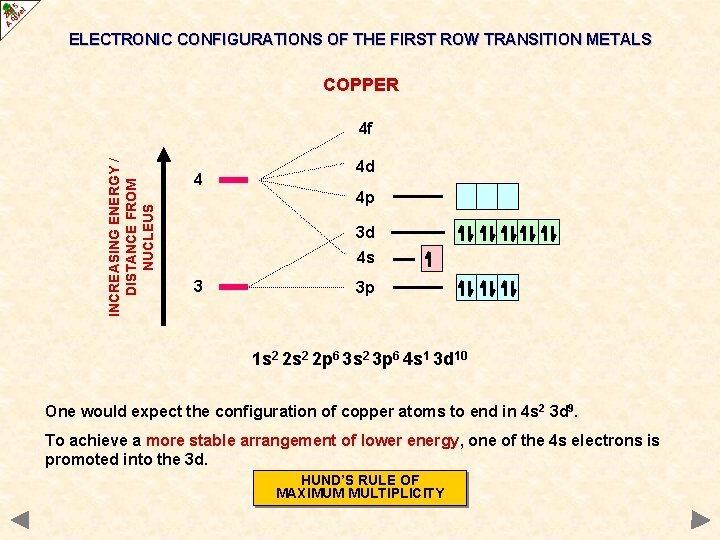
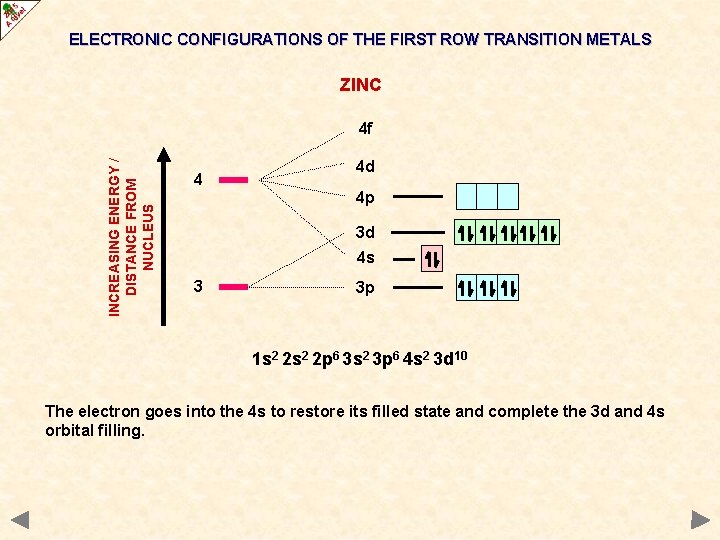
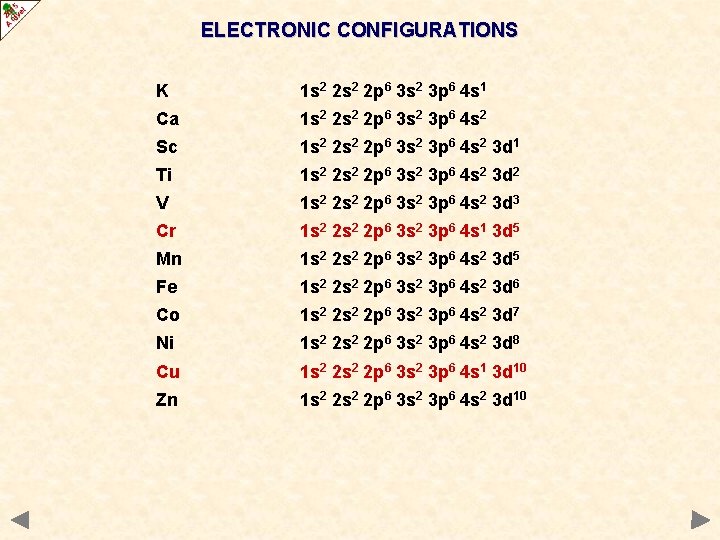
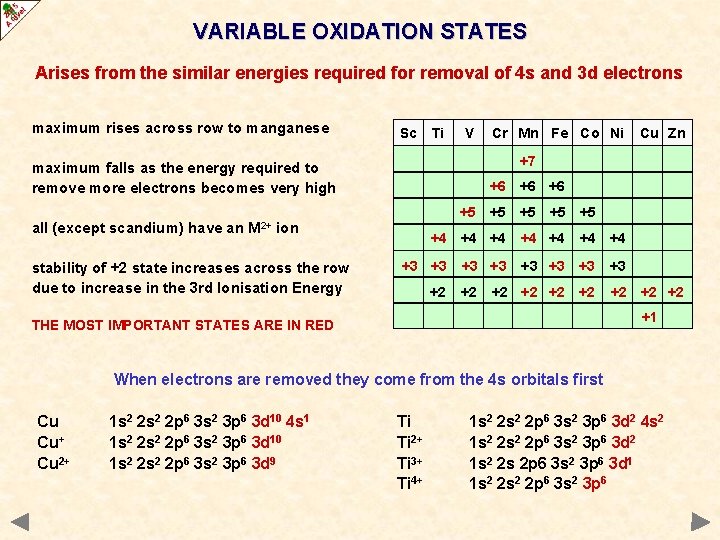
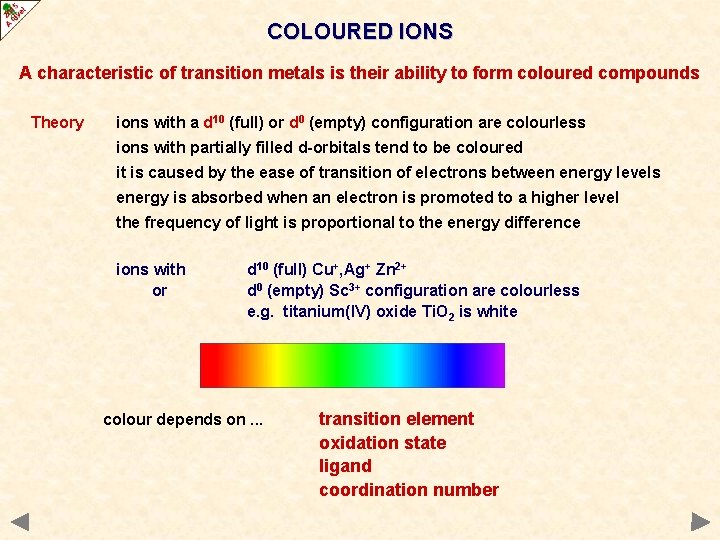
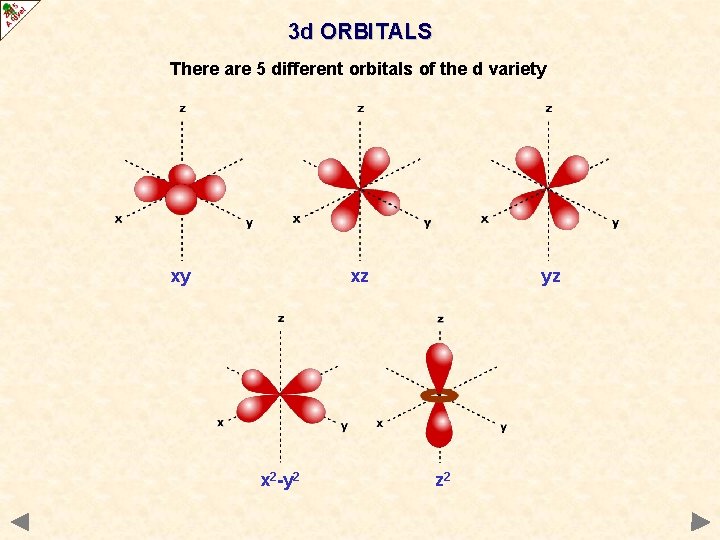
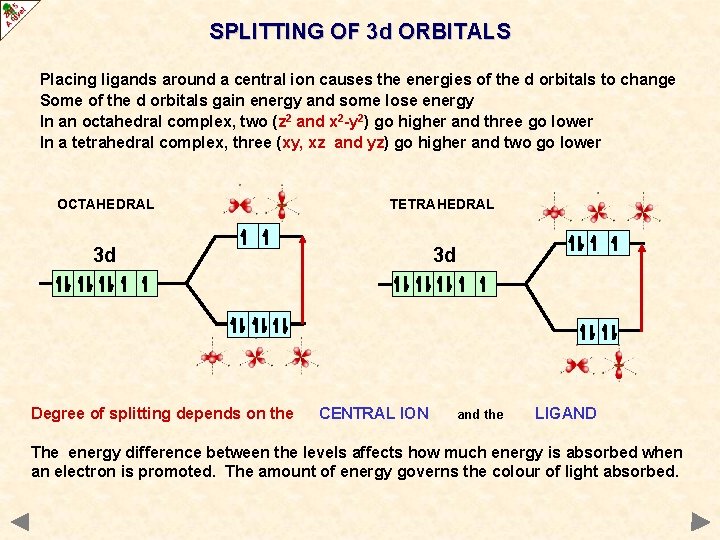
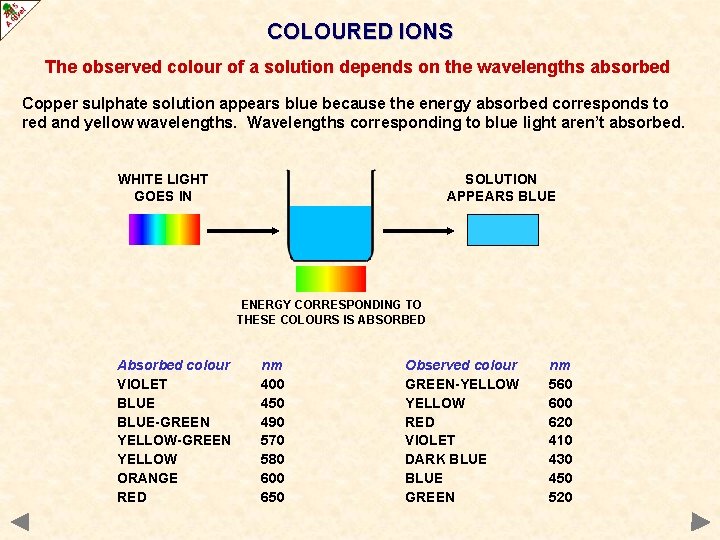
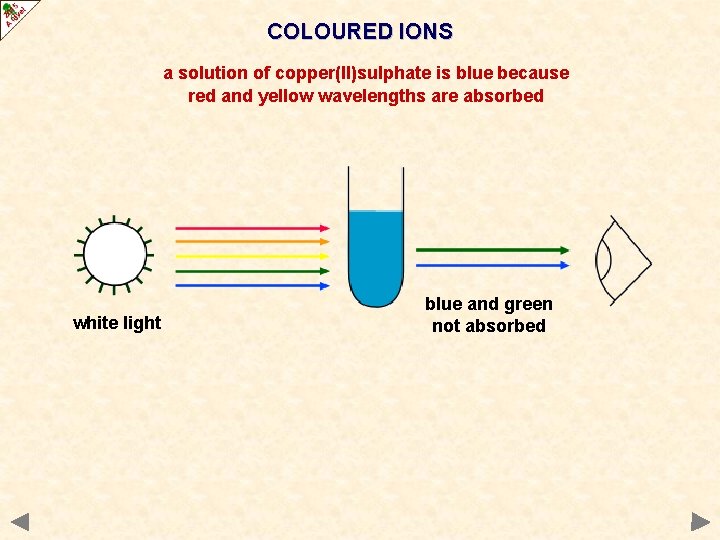
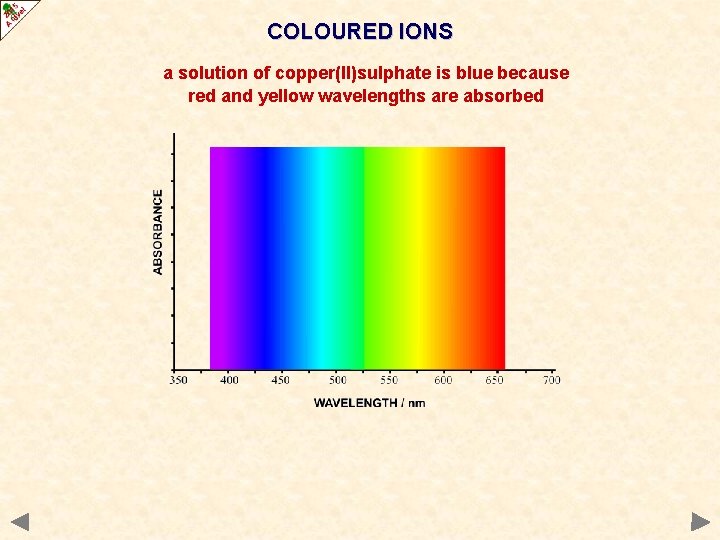
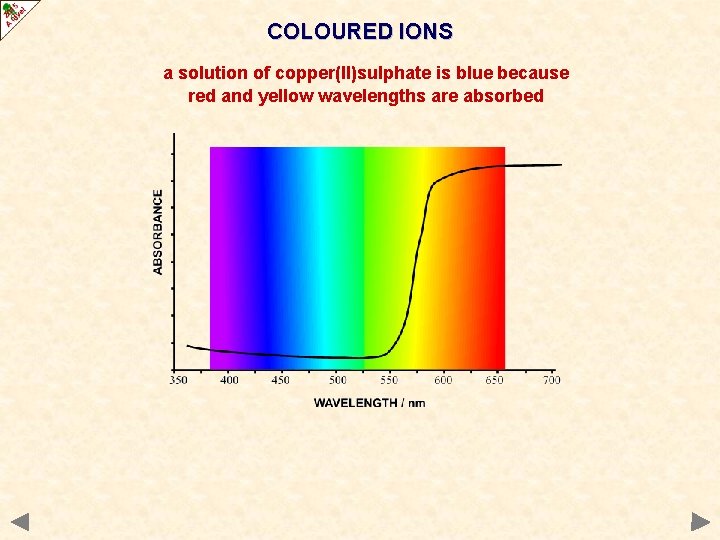
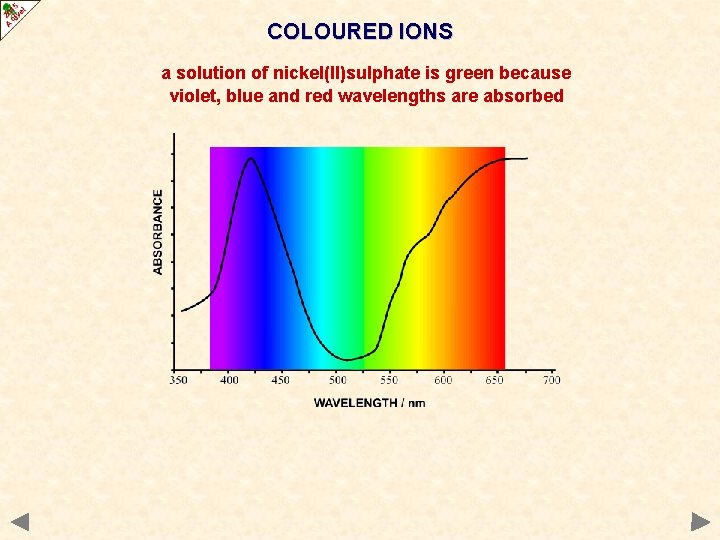
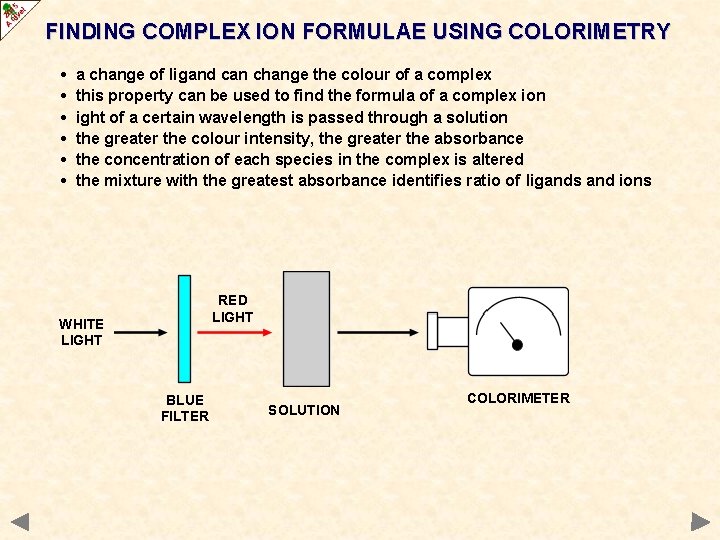
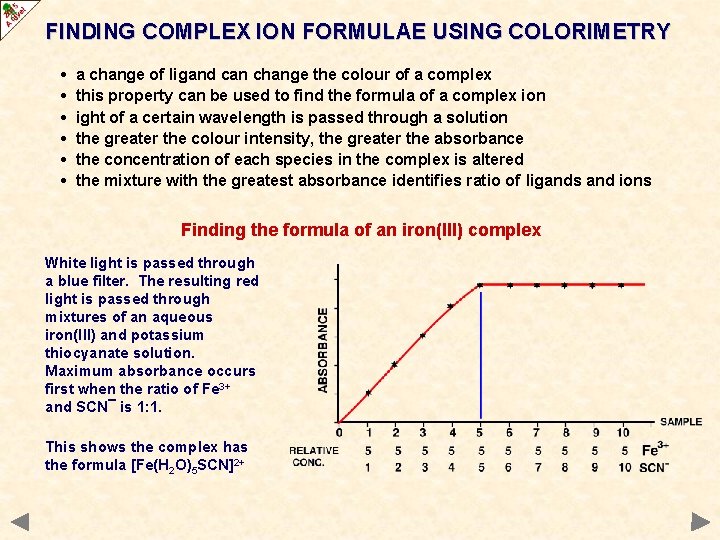
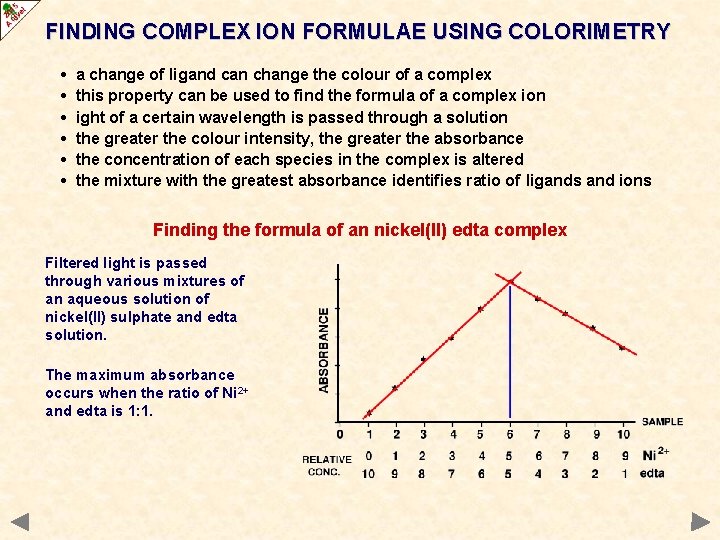
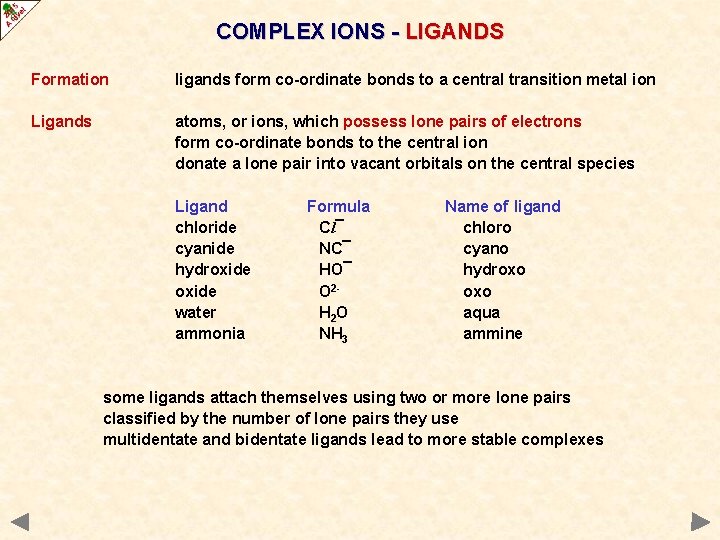
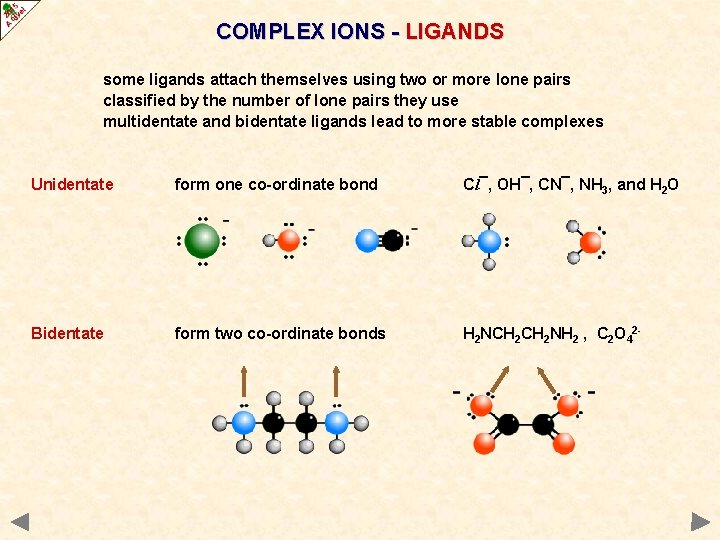
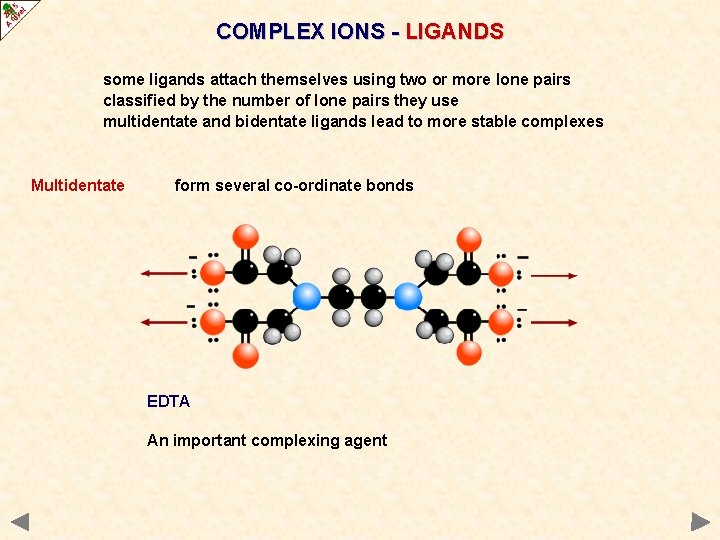
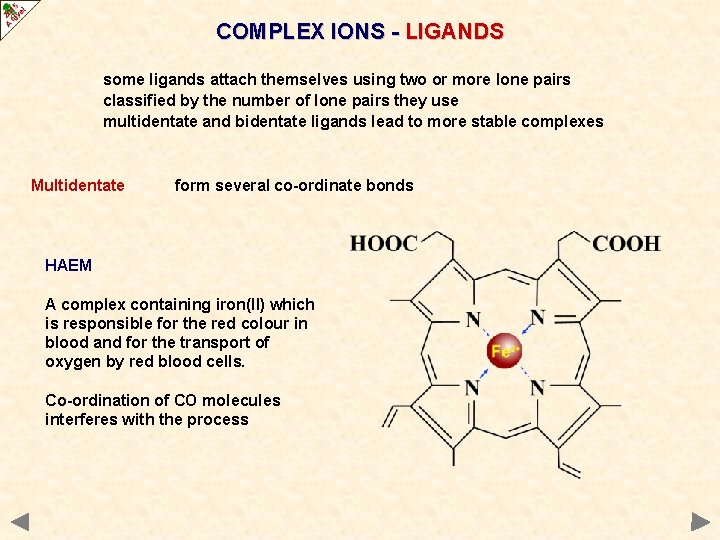
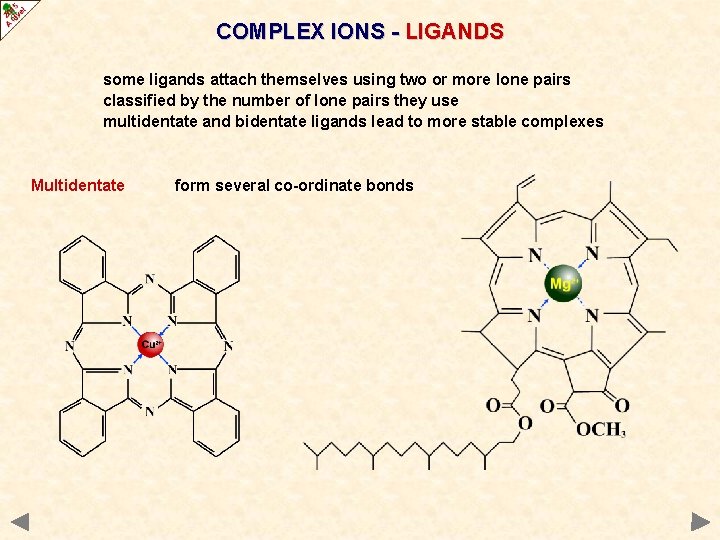
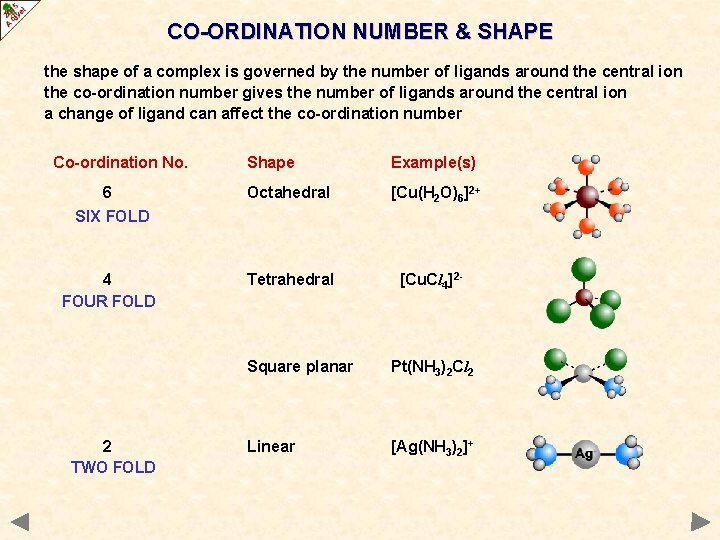
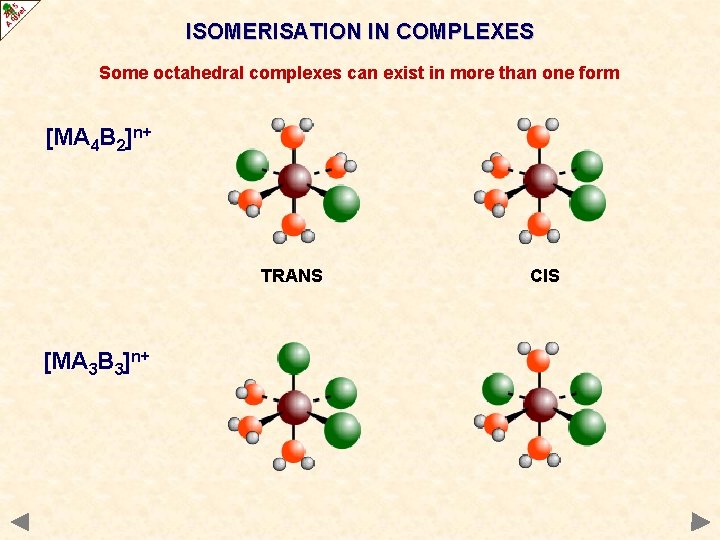
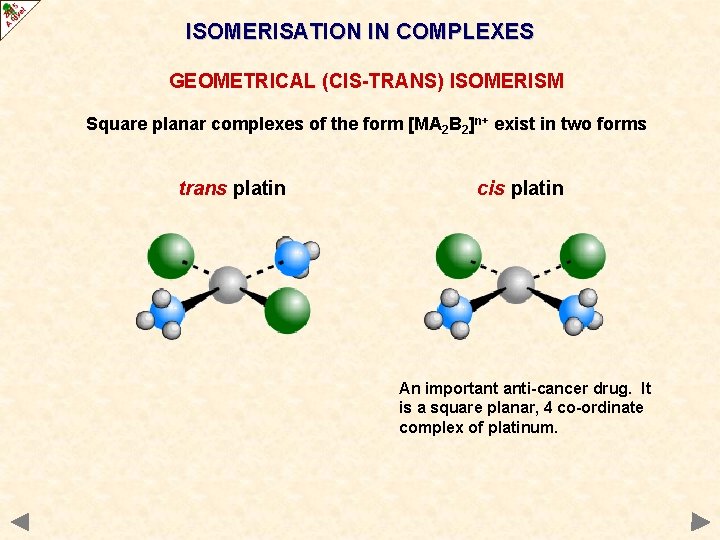
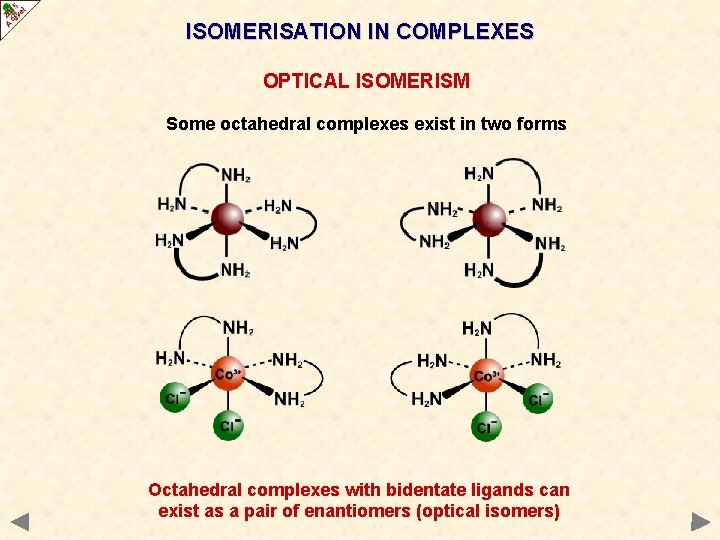
![ISOMERISATION IN COMPLEXES OPTICAL ISOMERISM AND GEOMETRICAL ISOMERSIM The complex ion [Co(en)2 Cl 2]+ ISOMERISATION IN COMPLEXES OPTICAL ISOMERISM AND GEOMETRICAL ISOMERSIM The complex ion [Co(en)2 Cl 2]+](https://slidetodoc.com/presentation_image_h/361e6347eb1946d07d7ff4d7d6d4a521/image-41.jpg)
![ISOMERISATION IN COMPLEXES OPTICAL ISOMERISM AND GEOMETRICAL ISOMERSIM The complex ion [Co(en)2 Cl 2]+ ISOMERISATION IN COMPLEXES OPTICAL ISOMERISM AND GEOMETRICAL ISOMERSIM The complex ion [Co(en)2 Cl 2]+](https://slidetodoc.com/presentation_image_h/361e6347eb1946d07d7ff4d7d6d4a521/image-42.jpg)
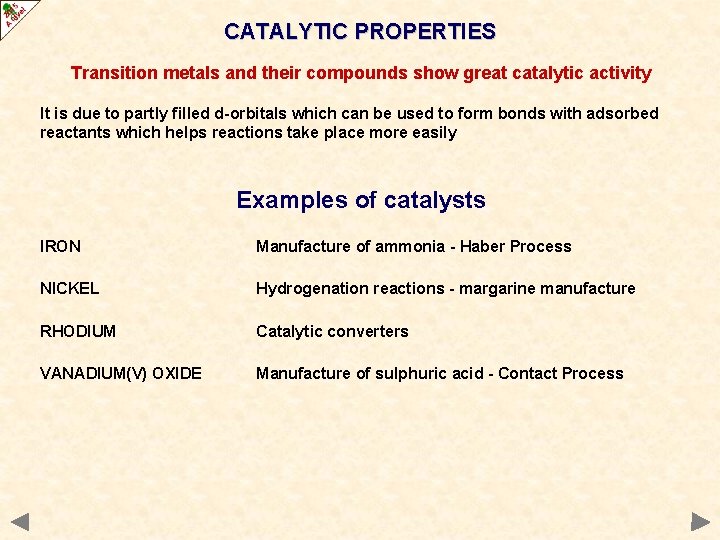
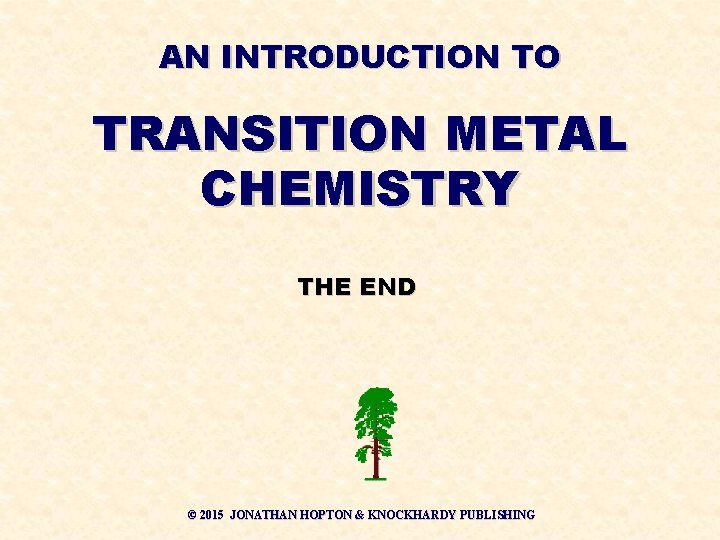
- Slides: 44
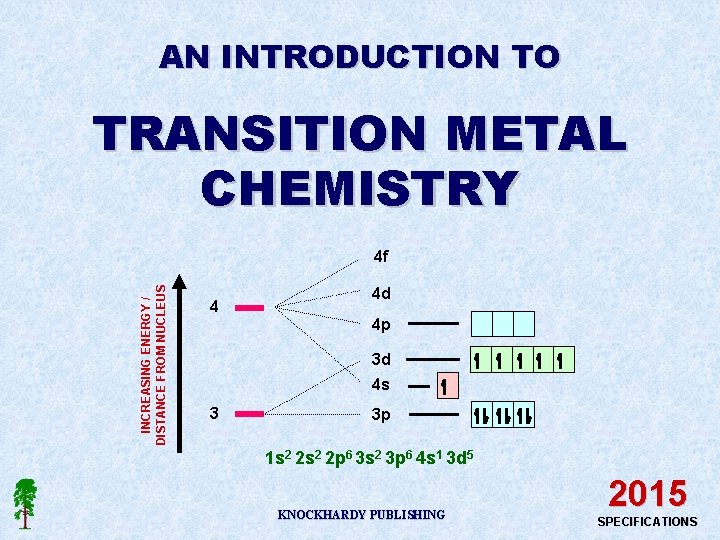
AN INTRODUCTION TO TRANSITION METAL CHEMISTRY INCREASING ENERGY / DISTANCE FROM NUCLEUS 4 f 4 4 d 4 p 3 d 4 s 3 3 p 1 s 2 2 p 6 3 s 2 3 p 6 4 s 1 3 d 5 KNOCKHARDY PUBLISHING 2015 SPECIFICATIONS
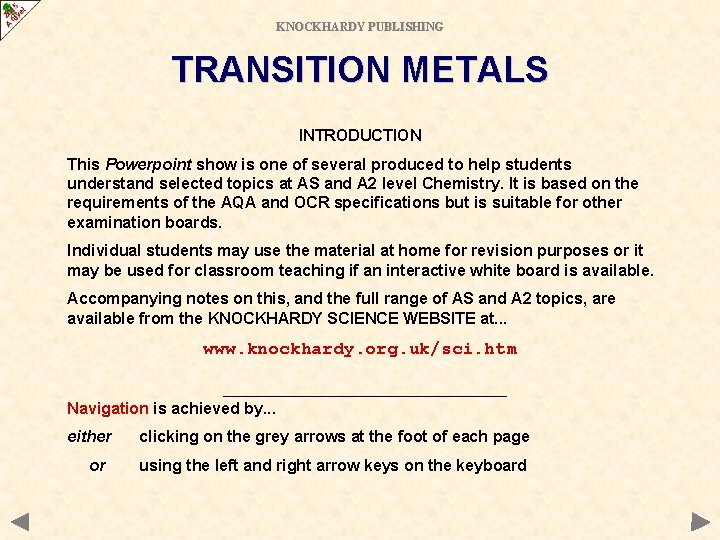
KNOCKHARDY PUBLISHING TRANSITION METALS INTRODUCTION This Powerpoint show is one of several produced to help students understand selected topics at AS and A 2 level Chemistry. It is based on the requirements of the AQA and OCR specifications but is suitable for other examination boards. Individual students may use the material at home for revision purposes or it may be used for classroom teaching if an interactive white board is available. Accompanying notes on this, and the full range of AS and A 2 topics, are available from the KNOCKHARDY SCIENCE WEBSITE at. . . www. knockhardy. org. uk/sci. htm Navigation is achieved by. . . either or clicking on the grey arrows at the foot of each page using the left and right arrow keys on the keyboard
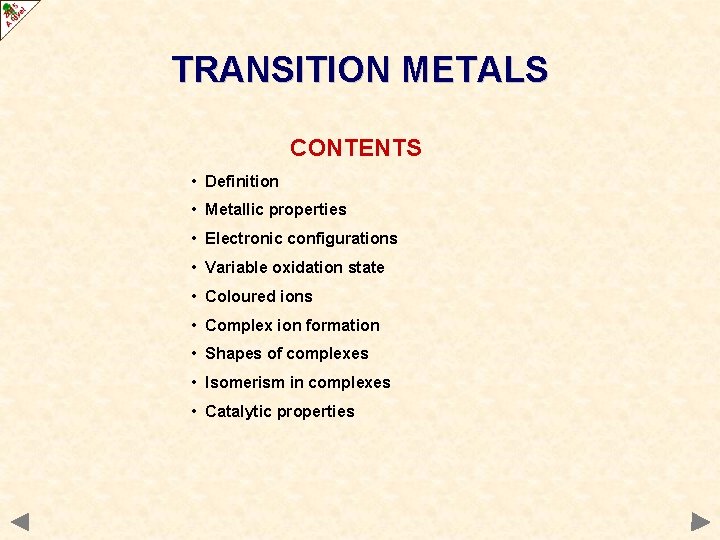
TRANSITION METALS CONTENTS • Definition • Metallic properties • Electronic configurations • Variable oxidation state • Coloured ions • Complex ion formation • Shapes of complexes • Isomerism in complexes • Catalytic properties
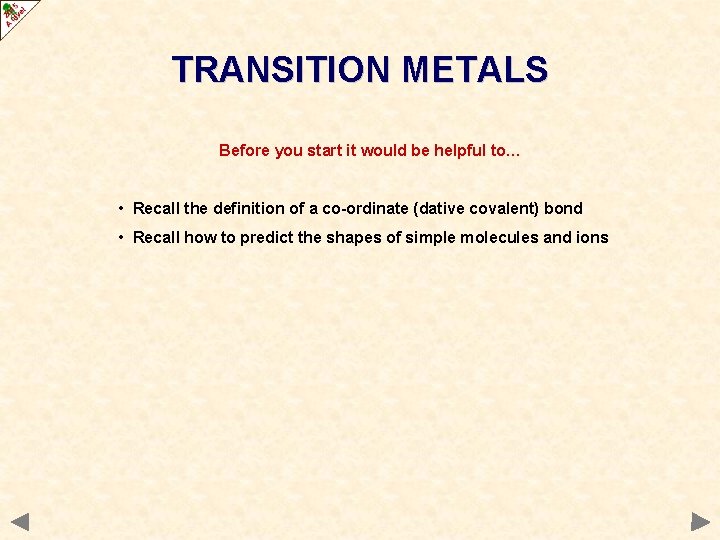
TRANSITION METALS Before you start it would be helpful to… • Recall the definition of a co-ordinate (dative covalent) bond • Recall how to predict the shapes of simple molecules and ions
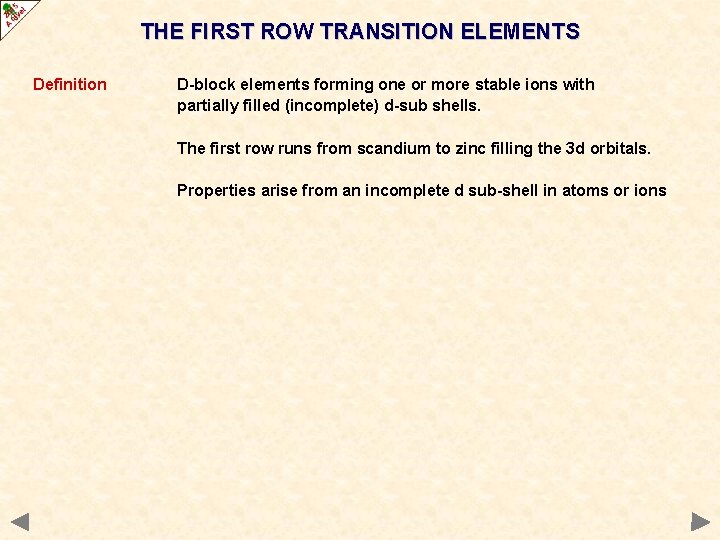
THE FIRST ROW TRANSITION ELEMENTS Definition D-block elements forming one or more stable ions with partially filled (incomplete) d-sub shells. The first row runs from scandium to zinc filling the 3 d orbitals. Properties arise from an incomplete d sub-shell in atoms or ions
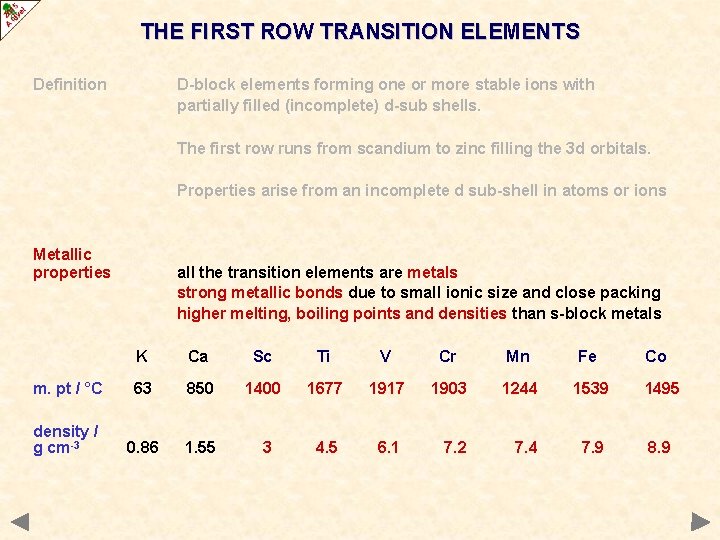
THE FIRST ROW TRANSITION ELEMENTS Definition D-block elements forming one or more stable ions with partially filled (incomplete) d-sub shells. The first row runs from scandium to zinc filling the 3 d orbitals. Properties arise from an incomplete d sub-shell in atoms or ions Metallic properties all the transition elements are metals strong metallic bonds due to small ionic size and close packing higher melting, boiling points and densities than s-block metals K Ca Sc Ti V Cr Mn Fe Co m. pt / °C 63 850 1400 1677 1917 1903 1244 1539 1495 density / g cm-3 0. 86 1. 55 3 4. 5 6. 1 7. 2 7. 4 7. 9 8. 9
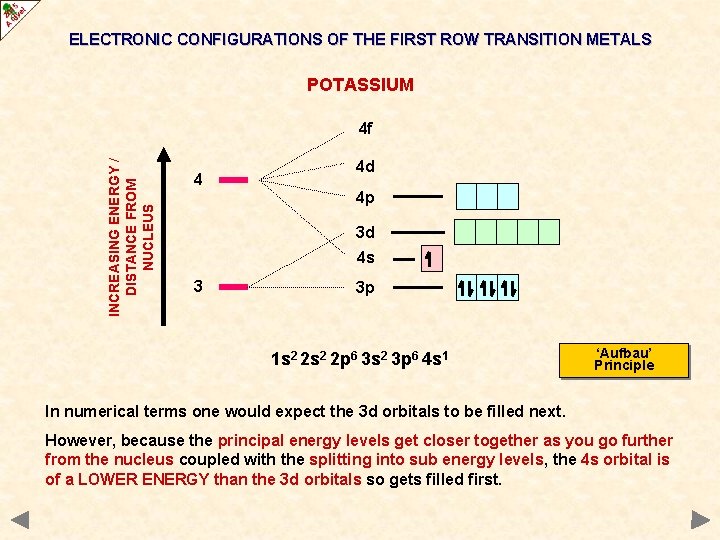
ELECTRONIC CONFIGURATIONS OF THE FIRST ROW TRANSITION METALS POTASSIUM INCREASING ENERGY / DISTANCE FROM NUCLEUS 4 f 4 4 d 4 p 3 d 4 s 3 3 p 1 s 2 2 p 6 3 s 2 3 p 6 4 s 1 ‘Aufbau’ Principle In numerical terms one would expect the 3 d orbitals to be filled next. However, because the principal energy levels get closer together as you go further from the nucleus coupled with the splitting into sub energy levels, the 4 s orbital is of a LOWER ENERGY than the 3 d orbitals so gets filled first.
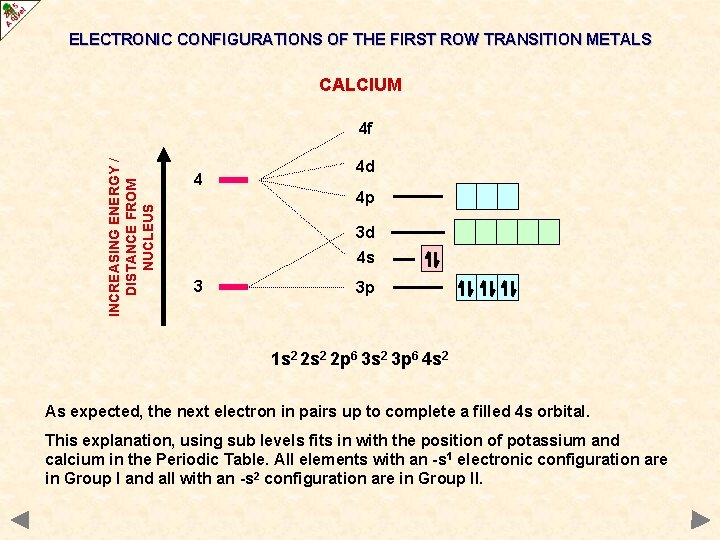
ELECTRONIC CONFIGURATIONS OF THE FIRST ROW TRANSITION METALS CALCIUM INCREASING ENERGY / DISTANCE FROM NUCLEUS 4 f 4 4 d 4 p 3 d 4 s 3 3 p 1 s 2 2 p 6 3 s 2 3 p 6 4 s 2 As expected, the next electron in pairs up to complete a filled 4 s orbital. This explanation, using sub levels fits in with the position of potassium and calcium in the Periodic Table. All elements with an -s 1 electronic configuration are in Group I and all with an -s 2 configuration are in Group II.
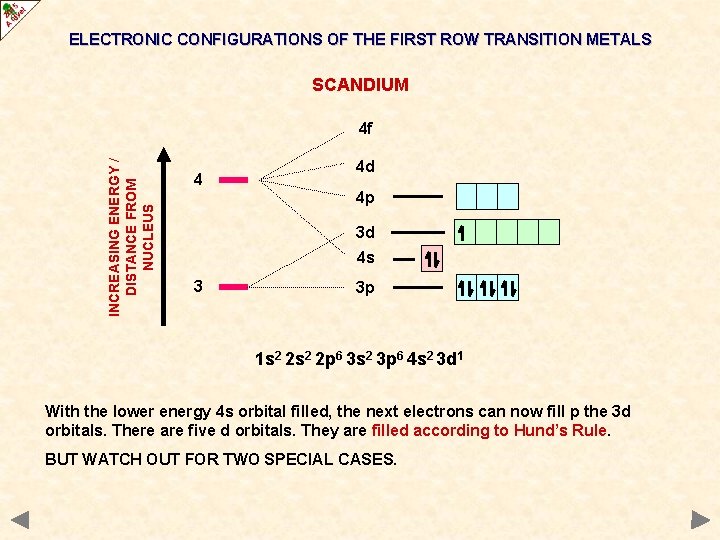
ELECTRONIC CONFIGURATIONS OF THE FIRST ROW TRANSITION METALS SCANDIUM INCREASING ENERGY / DISTANCE FROM NUCLEUS 4 f 4 4 d 4 p 3 d 4 s 3 3 p 1 s 2 2 p 6 3 s 2 3 p 6 4 s 2 3 d 1 With the lower energy 4 s orbital filled, the next electrons can now fill p the 3 d orbitals. There are five d orbitals. They are filled according to Hund’s Rule. BUT WATCH OUT FOR TWO SPECIAL CASES.
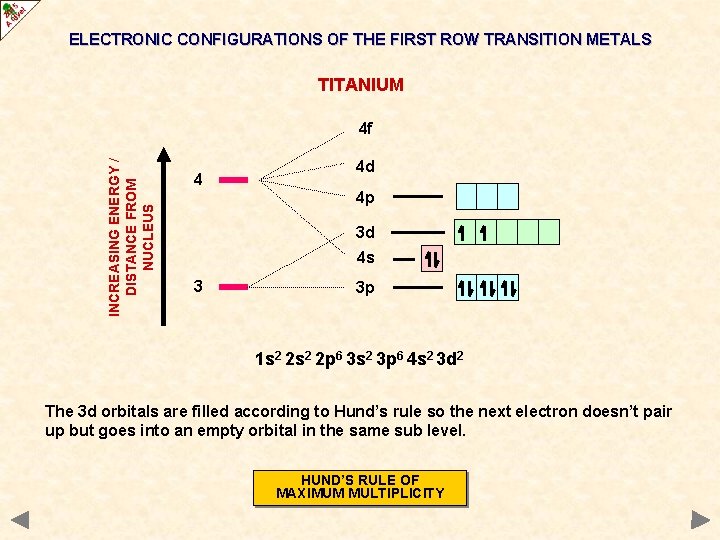
ELECTRONIC CONFIGURATIONS OF THE FIRST ROW TRANSITION METALS TITANIUM INCREASING ENERGY / DISTANCE FROM NUCLEUS 4 f 4 4 d 4 p 3 d 4 s 3 3 p 1 s 2 2 p 6 3 s 2 3 p 6 4 s 2 3 d 2 The 3 d orbitals are filled according to Hund’s rule so the next electron doesn’t pair up but goes into an empty orbital in the same sub level. HUND’S RULE OF MAXIMUM MULTIPLICITY
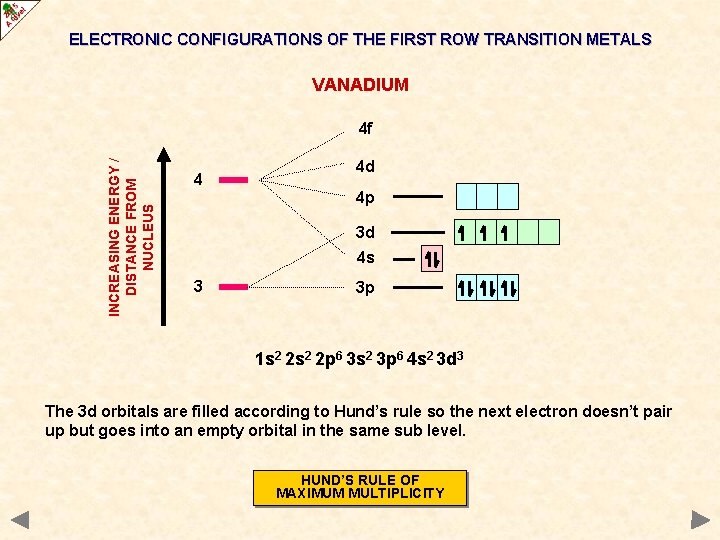
ELECTRONIC CONFIGURATIONS OF THE FIRST ROW TRANSITION METALS VANADIUM INCREASING ENERGY / DISTANCE FROM NUCLEUS 4 f 4 4 d 4 p 3 d 4 s 3 3 p 1 s 2 2 p 6 3 s 2 3 p 6 4 s 2 3 d 3 The 3 d orbitals are filled according to Hund’s rule so the next electron doesn’t pair up but goes into an empty orbital in the same sub level. HUND’S RULE OF MAXIMUM MULTIPLICITY
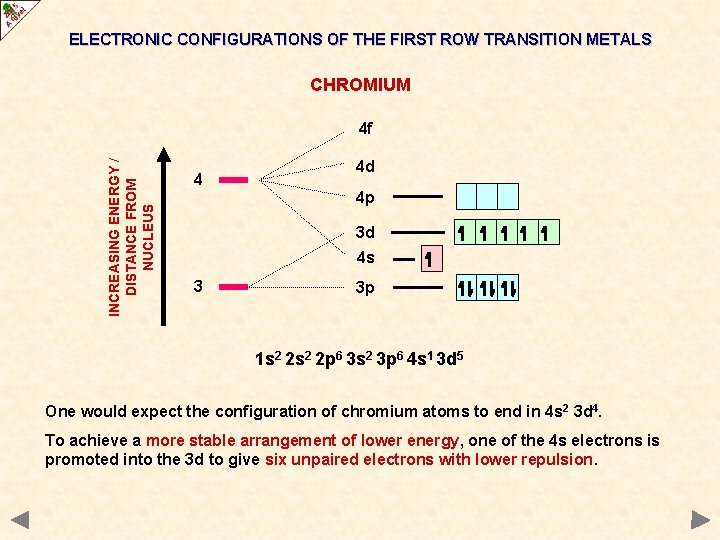
ELECTRONIC CONFIGURATIONS OF THE FIRST ROW TRANSITION METALS CHROMIUM INCREASING ENERGY / DISTANCE FROM NUCLEUS 4 f 4 4 d 4 p 3 d 4 s 3 3 p 1 s 2 2 p 6 3 s 2 3 p 6 4 s 1 3 d 5 One would expect the configuration of chromium atoms to end in 4 s 2 3 d 4. To achieve a more stable arrangement of lower energy, one of the 4 s electrons is promoted into the 3 d to give six unpaired electrons with lower repulsion.
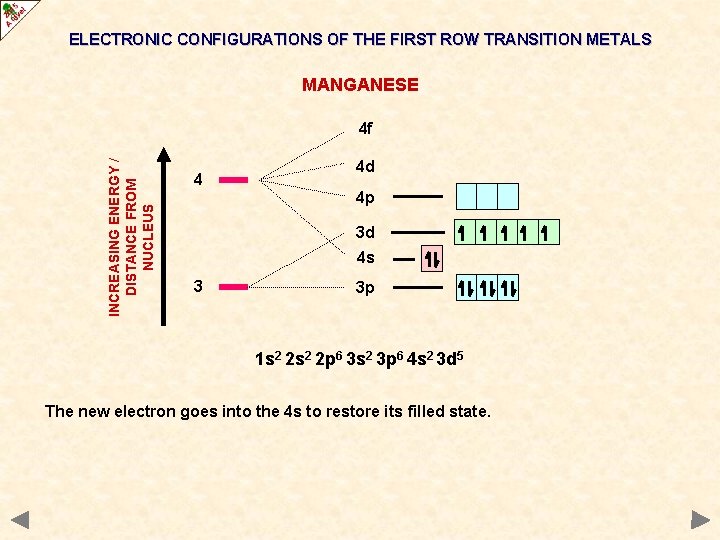
ELECTRONIC CONFIGURATIONS OF THE FIRST ROW TRANSITION METALS MANGANESE INCREASING ENERGY / DISTANCE FROM NUCLEUS 4 f 4 4 d 4 p 3 d 4 s 3 3 p 1 s 2 2 p 6 3 s 2 3 p 6 4 s 2 3 d 5 The new electron goes into the 4 s to restore its filled state.
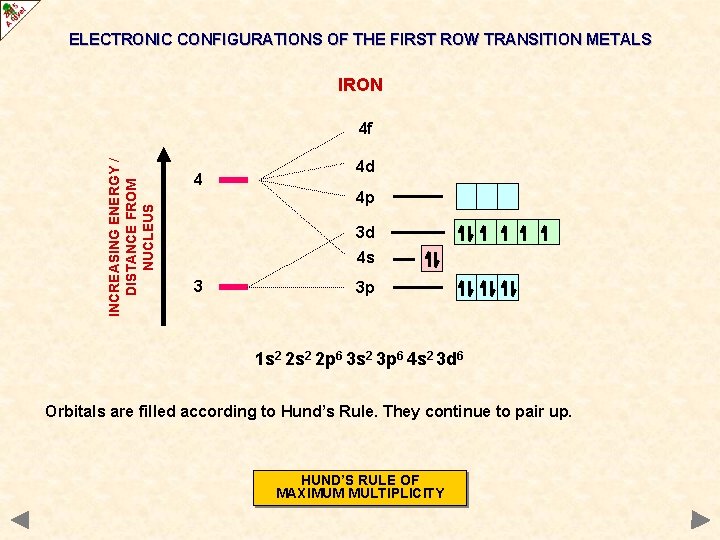
ELECTRONIC CONFIGURATIONS OF THE FIRST ROW TRANSITION METALS IRON INCREASING ENERGY / DISTANCE FROM NUCLEUS 4 f 4 4 d 4 p 3 d 4 s 3 3 p 1 s 2 2 p 6 3 s 2 3 p 6 4 s 2 3 d 6 Orbitals are filled according to Hund’s Rule. They continue to pair up. HUND’S RULE OF MAXIMUM MULTIPLICITY
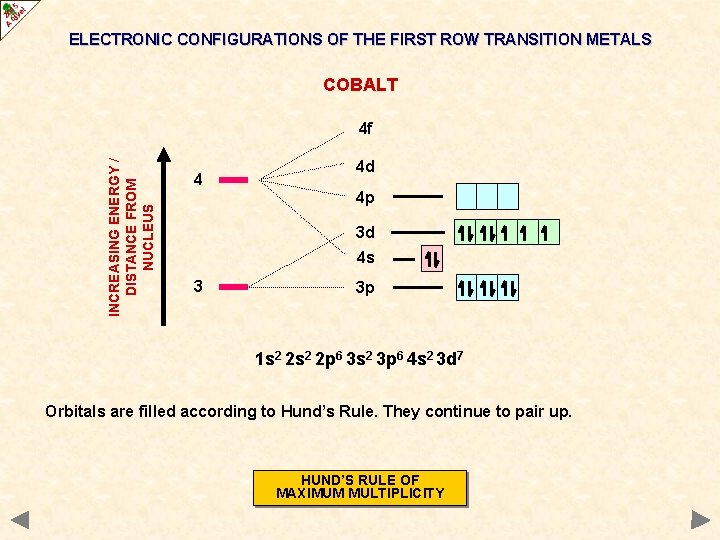
ELECTRONIC CONFIGURATIONS OF THE FIRST ROW TRANSITION METALS COBALT INCREASING ENERGY / DISTANCE FROM NUCLEUS 4 f 4 4 d 4 p 3 d 4 s 3 3 p 1 s 2 2 p 6 3 s 2 3 p 6 4 s 2 3 d 7 Orbitals are filled according to Hund’s Rule. They continue to pair up. HUND’S RULE OF MAXIMUM MULTIPLICITY
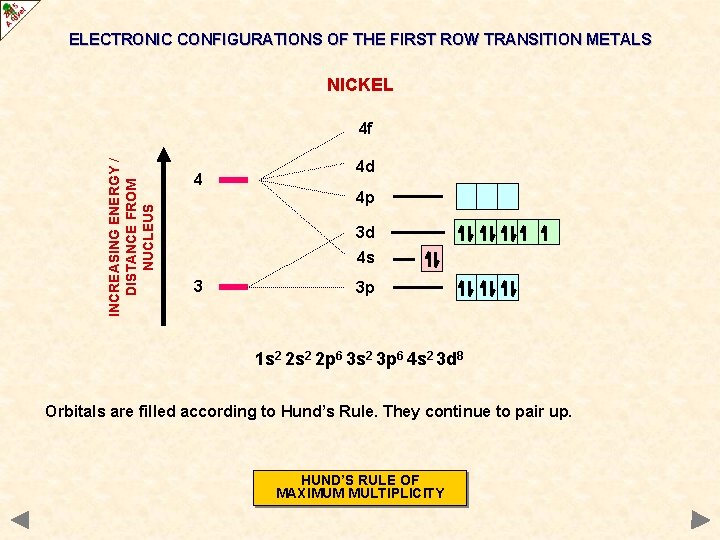
ELECTRONIC CONFIGURATIONS OF THE FIRST ROW TRANSITION METALS NICKEL INCREASING ENERGY / DISTANCE FROM NUCLEUS 4 f 4 4 d 4 p 3 d 4 s 3 3 p 1 s 2 2 p 6 3 s 2 3 p 6 4 s 2 3 d 8 Orbitals are filled according to Hund’s Rule. They continue to pair up. HUND’S RULE OF MAXIMUM MULTIPLICITY
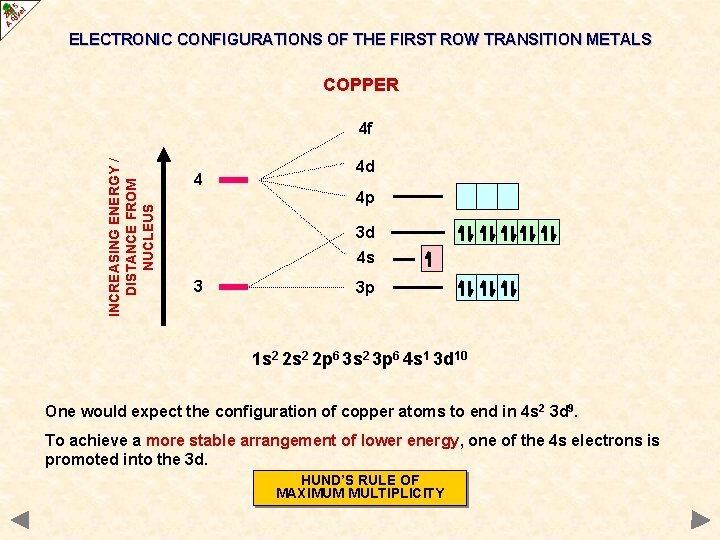
ELECTRONIC CONFIGURATIONS OF THE FIRST ROW TRANSITION METALS COPPER INCREASING ENERGY / DISTANCE FROM NUCLEUS 4 f 4 4 d 4 p 3 d 4 s 3 3 p 1 s 2 2 p 6 3 s 2 3 p 6 4 s 1 3 d 10 One would expect the configuration of copper atoms to end in 4 s 2 3 d 9. To achieve a more stable arrangement of lower energy, one of the 4 s electrons is promoted into the 3 d. HUND’S RULE OF MAXIMUM MULTIPLICITY
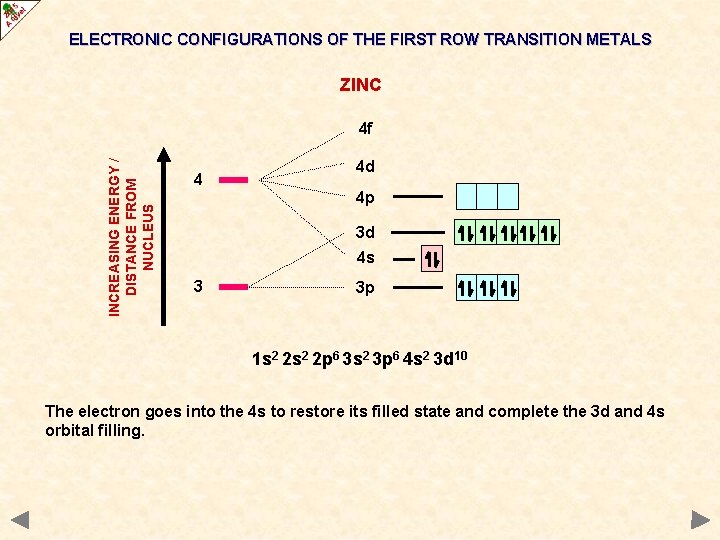
ELECTRONIC CONFIGURATIONS OF THE FIRST ROW TRANSITION METALS ZINC INCREASING ENERGY / DISTANCE FROM NUCLEUS 4 f 4 4 d 4 p 3 d 4 s 3 3 p 1 s 2 2 p 6 3 s 2 3 p 6 4 s 2 3 d 10 The electron goes into the 4 s to restore its filled state and complete the 3 d and 4 s orbital filling.
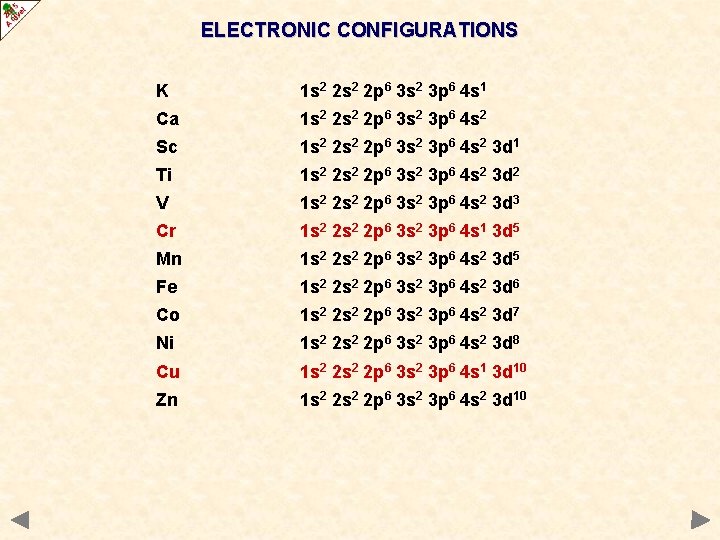
ELECTRONIC CONFIGURATIONS K 1 s 2 2 p 6 3 s 2 3 p 6 4 s 1 Ca 1 s 2 2 p 6 3 s 2 3 p 6 4 s 2 Sc 1 s 2 2 p 6 3 s 2 3 p 6 4 s 2 3 d 1 Ti 1 s 2 2 p 6 3 s 2 3 p 6 4 s 2 3 d 2 V 1 s 2 2 p 6 3 s 2 3 p 6 4 s 2 3 d 3 Cr 1 s 2 2 p 6 3 s 2 3 p 6 4 s 1 3 d 5 Mn 1 s 2 2 p 6 3 s 2 3 p 6 4 s 2 3 d 5 Fe 1 s 2 2 p 6 3 s 2 3 p 6 4 s 2 3 d 6 Co 1 s 2 2 p 6 3 s 2 3 p 6 4 s 2 3 d 7 Ni 1 s 2 2 p 6 3 s 2 3 p 6 4 s 2 3 d 8 Cu 1 s 2 2 p 6 3 s 2 3 p 6 4 s 1 3 d 10 Zn 1 s 2 2 p 6 3 s 2 3 p 6 4 s 2 3 d 10
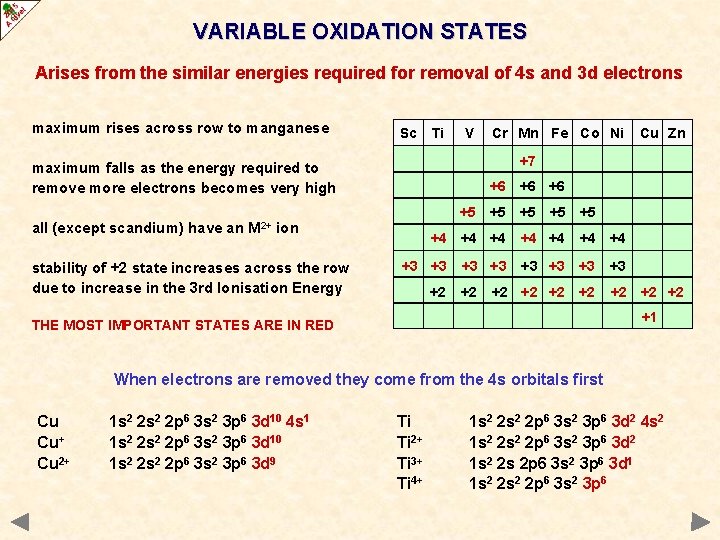
VARIABLE OXIDATION STATES Arises from the similar energies required for removal of 4 s and 3 d electrons maximum rises across row to manganese Sc Ti V +5 all (except scandium) have an ion stability of +2 state increases across the row due to increase in the 3 rd Ionisation Energy Cu Zn +7 maximum falls as the energy required to remove more electrons becomes very high M 2+ Cr Mn Fe Co Ni +6 +6 +6 +5 +5 +4 +4 +3 +3 +2 +2 +2 +1 THE MOST IMPORTANT STATES ARE IN RED When electrons are removed they come from the 4 s orbitals first Cu Cu+ Cu 2+ 1 s 2 2 p 6 3 s 2 3 p 6 3 d 10 4 s 1 1 s 2 2 p 6 3 s 2 3 p 6 3 d 10 1 s 2 2 p 6 3 s 2 3 p 6 3 d 9 Ti Ti 2+ Ti 3+ Ti 4+ 1 s 2 2 p 6 3 s 2 3 p 6 3 d 2 4 s 2 1 s 2 2 p 6 3 s 2 3 p 6 3 d 2 1 s 2 2 s 2 p 6 3 s 2 3 p 6 3 d 1 1 s 2 2 p 6 3 s 2 3 p 6
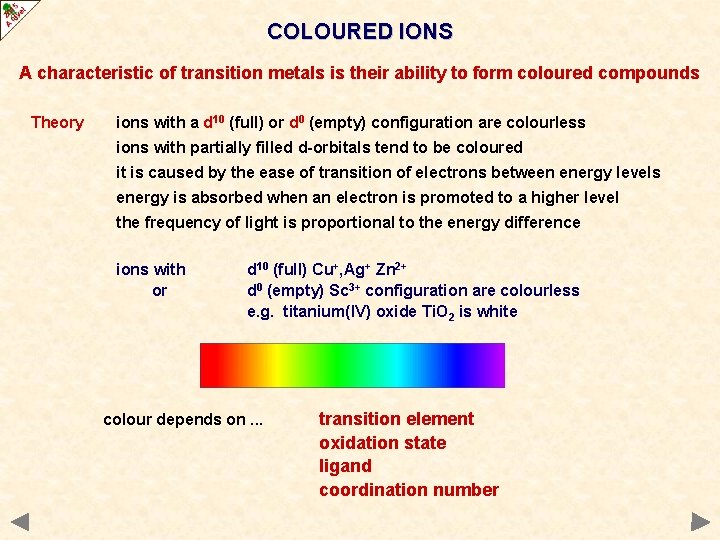
COLOURED IONS A characteristic of transition metals is their ability to form coloured compounds Theory ions with a d 10 (full) or d 0 (empty) configuration are colourless ions with partially filled d-orbitals tend to be coloured it is caused by the ease of transition of electrons between energy levels energy is absorbed when an electron is promoted to a higher level the frequency of light is proportional to the energy difference ions with or d 10 (full) Cu+, Ag+ Zn 2+ d 0 (empty) Sc 3+ configuration are colourless e. g. titanium(IV) oxide Ti. O 2 is white colour depends on. . . transition element oxidation state ligand coordination number
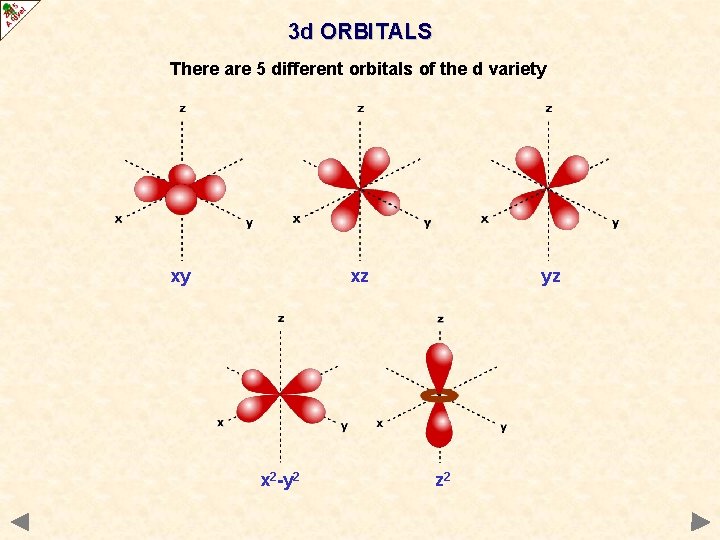
3 d ORBITALS There are 5 different orbitals of the d variety xy xz x 2 -y 2 yz z 2
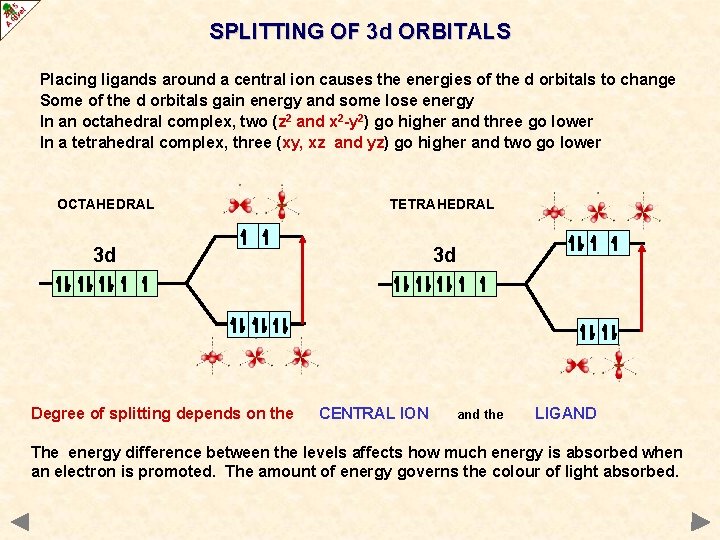
SPLITTING OF 3 d ORBITALS Placing ligands around a central ion causes the energies of the d orbitals to change Some of the d orbitals gain energy and some lose energy In an octahedral complex, two (z 2 and x 2 -y 2) go higher and three go lower In a tetrahedral complex, three (xy, xz and yz) go higher and two go lower OCTAHEDRAL TETRAHEDRAL 3 d 3 d Degree of splitting depends on the CENTRAL ION and the LIGAND The energy difference between the levels affects how much energy is absorbed when an electron is promoted. The amount of energy governs the colour of light absorbed.
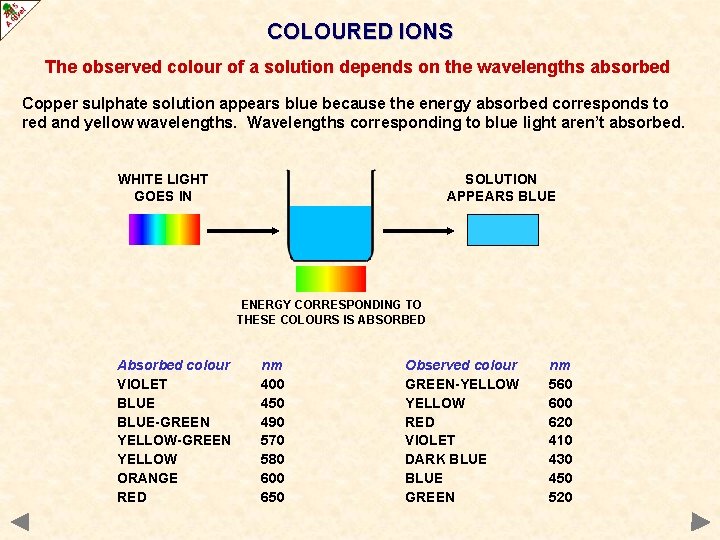
COLOURED IONS The observed colour of a solution depends on the wavelengths absorbed Copper sulphate solution appears blue because the energy absorbed corresponds to red and yellow wavelengths. Wavelengths corresponding to blue light aren’t absorbed. WHITE LIGHT GOES IN SOLUTION APPEARS BLUE ENERGY CORRESPONDING TO THESE COLOURS IS ABSORBED Absorbed colour VIOLET BLUE-GREEN YELLOW ORANGE RED nm 400 450 490 570 580 600 650 Observed colour GREEN-YELLOW RED VIOLET DARK BLUE GREEN nm 560 600 620 410 430 450 520
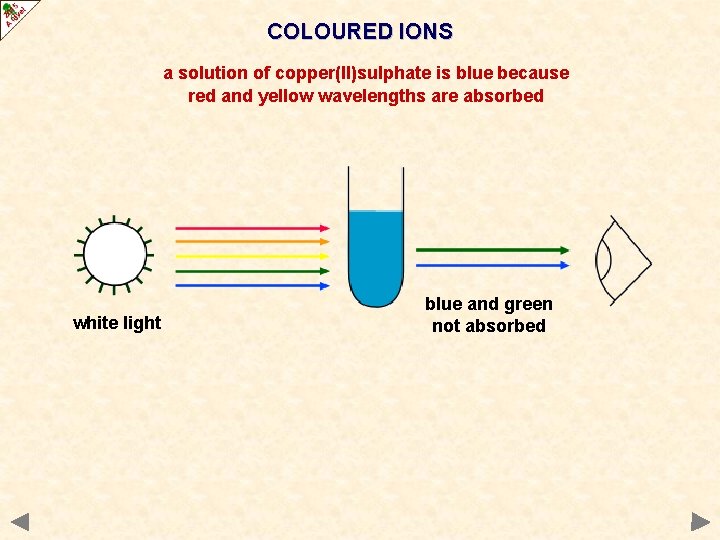
COLOURED IONS a solution of copper(II)sulphate is blue because red and yellow wavelengths are absorbed white light blue and green not absorbed
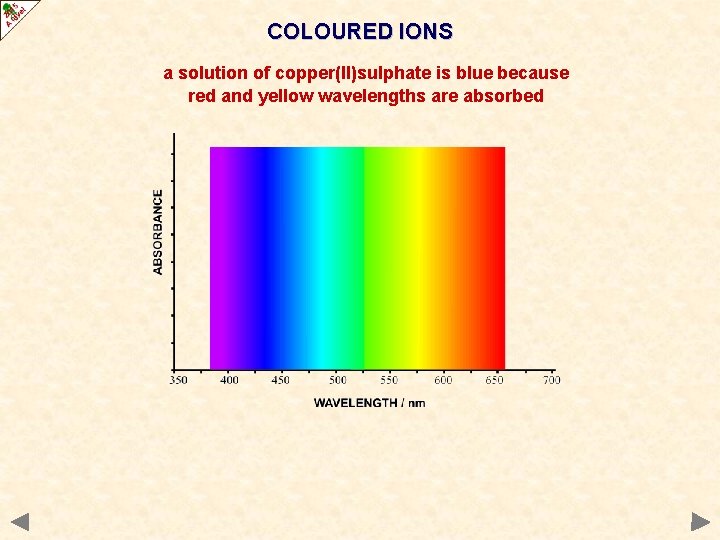
COLOURED IONS a solution of copper(II)sulphate is blue because red and yellow wavelengths are absorbed
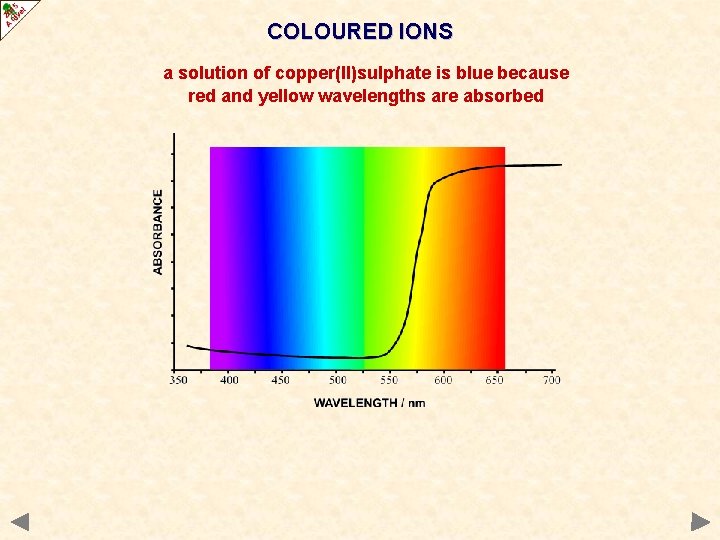
COLOURED IONS a solution of copper(II)sulphate is blue because red and yellow wavelengths are absorbed
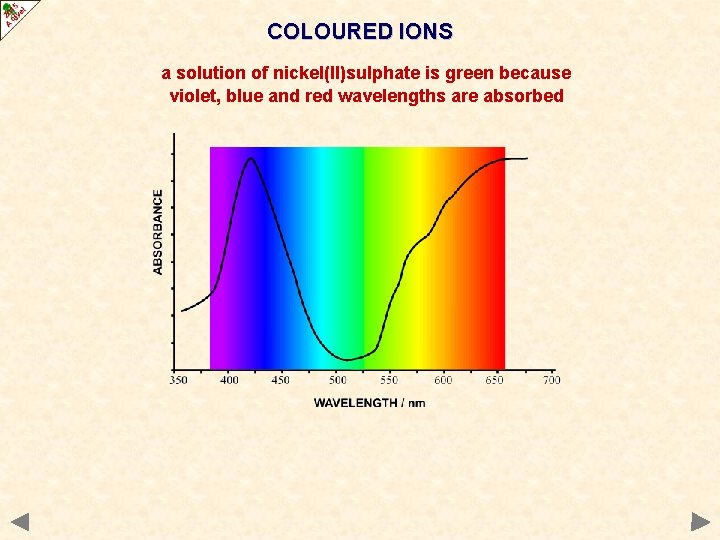
COLOURED IONS a solution of nickel(II)sulphate is green because violet, blue and red wavelengths are absorbed
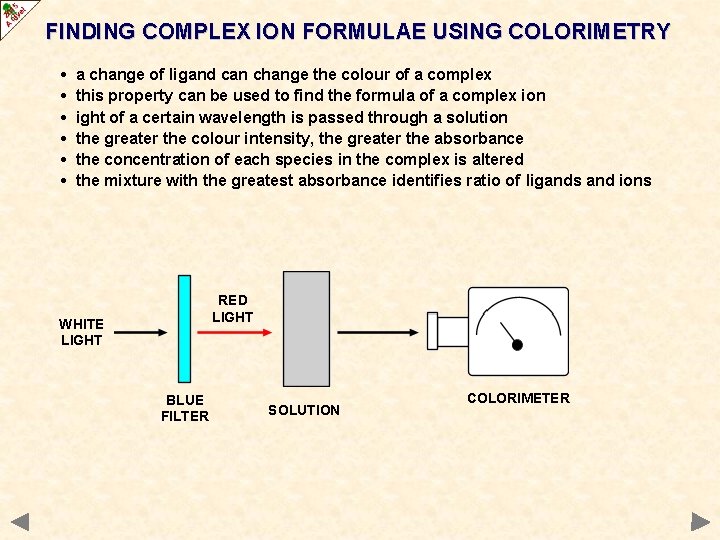
FINDING COMPLEX ION FORMULAE USING COLORIMETRY • • • a change of ligand can change the colour of a complex this property can be used to find the formula of a complex ion ight of a certain wavelength is passed through a solution the greater the colour intensity, the greater the absorbance the concentration of each species in the complex is altered the mixture with the greatest absorbance identifies ratio of ligands and ions RED LIGHT WHITE LIGHT BLUE FILTER SOLUTION COLORIMETER
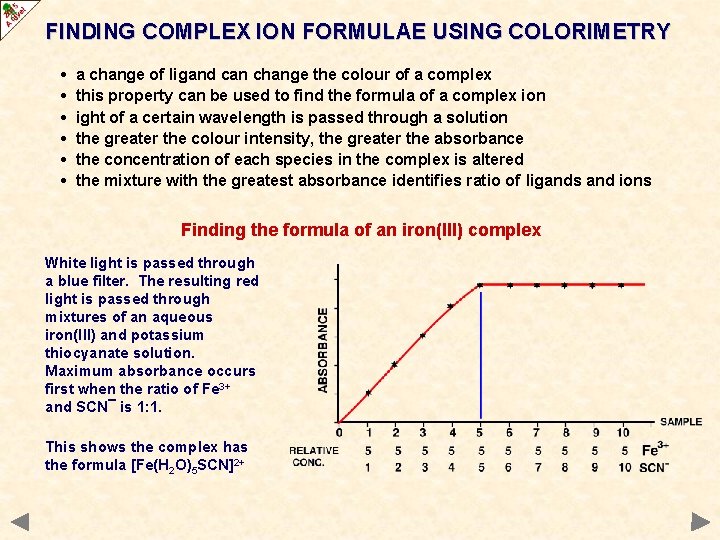
FINDING COMPLEX ION FORMULAE USING COLORIMETRY • • • a change of ligand can change the colour of a complex this property can be used to find the formula of a complex ion ight of a certain wavelength is passed through a solution the greater the colour intensity, the greater the absorbance the concentration of each species in the complex is altered the mixture with the greatest absorbance identifies ratio of ligands and ions Finding the formula of an iron(III) complex White light is passed through a blue filter. The resulting red light is passed through mixtures of an aqueous iron(III) and potassium thiocyanate solution. Maximum absorbance occurs first when the ratio of Fe 3+ and SCN¯ is 1: 1. This shows the complex has the formula [Fe(H 2 O)5 SCN]2+
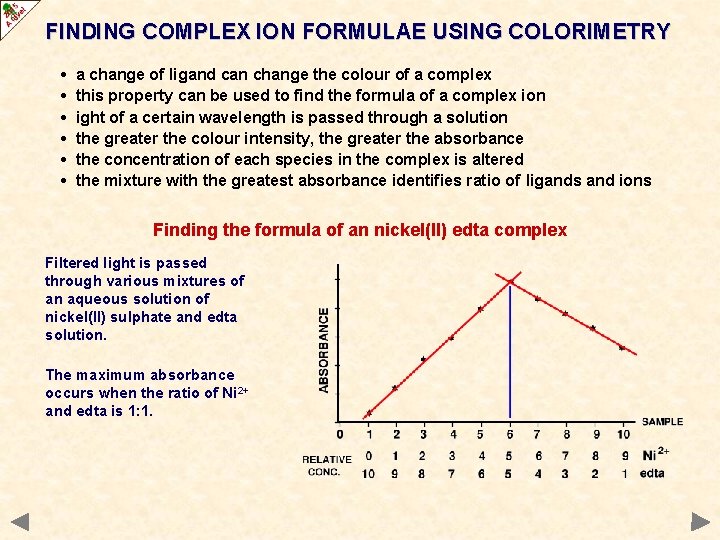
FINDING COMPLEX ION FORMULAE USING COLORIMETRY • • • a change of ligand can change the colour of a complex this property can be used to find the formula of a complex ion ight of a certain wavelength is passed through a solution the greater the colour intensity, the greater the absorbance the concentration of each species in the complex is altered the mixture with the greatest absorbance identifies ratio of ligands and ions Finding the formula of an nickel(II) edta complex Filtered light is passed through various mixtures of an aqueous solution of nickel(II) sulphate and edta solution. The maximum absorbance occurs when the ratio of Ni 2+ and edta is 1: 1.
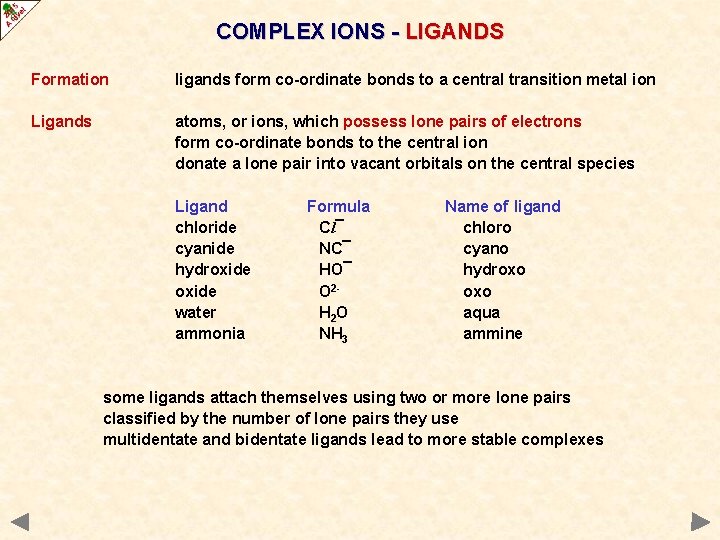
COMPLEX IONS - LIGANDS Formation ligands form co-ordinate bonds to a central transition metal ion Ligands atoms, or ions, which possess lone pairs of electrons form co-ordinate bonds to the central ion donate a lone pair into vacant orbitals on the central species Ligand chloride cyanide hydroxide water ammonia Formula Cl¯ NC¯ HO¯ O 2 H 2 O NH 3 Name of ligand chloro cyano hydroxo aqua ammine some ligands attach themselves using two or more lone pairs classified by the number of lone pairs they use multidentate and bidentate ligands lead to more stable complexes
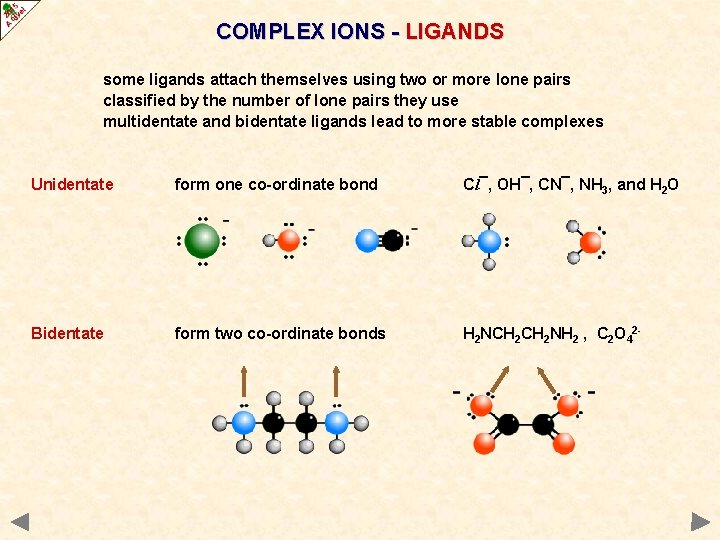
COMPLEX IONS - LIGANDS some ligands attach themselves using two or more lone pairs classified by the number of lone pairs they use multidentate and bidentate ligands lead to more stable complexes Unidentate form one co-ordinate bond Cl¯, OH¯, CN¯, NH 3, and H 2 O Bidentate form two co-ordinate bonds H 2 NCH 2 NH 2 , C 2 O 42 -
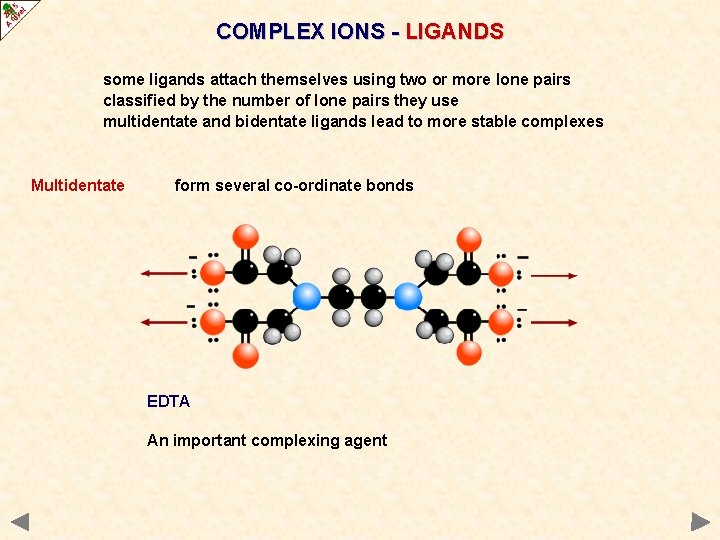
COMPLEX IONS - LIGANDS some ligands attach themselves using two or more lone pairs classified by the number of lone pairs they use multidentate and bidentate ligands lead to more stable complexes Multidentate form several co-ordinate bonds EDTA An important complexing agent
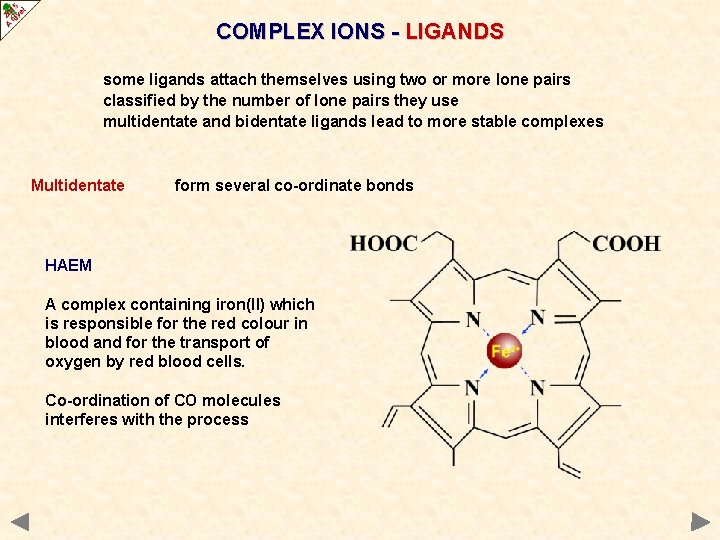
COMPLEX IONS - LIGANDS some ligands attach themselves using two or more lone pairs classified by the number of lone pairs they use multidentate and bidentate ligands lead to more stable complexes Multidentate form several co-ordinate bonds HAEM A complex containing iron(II) which is responsible for the red colour in blood and for the transport of oxygen by red blood cells. Co-ordination of CO molecules interferes with the process
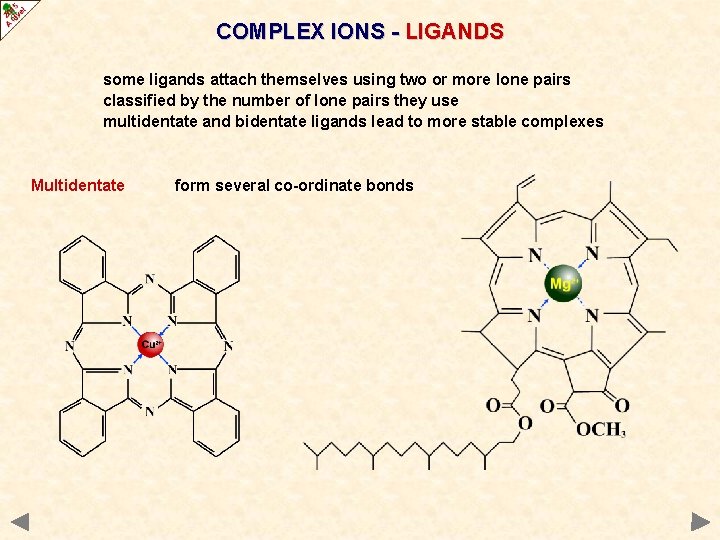
COMPLEX IONS - LIGANDS some ligands attach themselves using two or more lone pairs classified by the number of lone pairs they use multidentate and bidentate ligands lead to more stable complexes Multidentate form several co-ordinate bonds
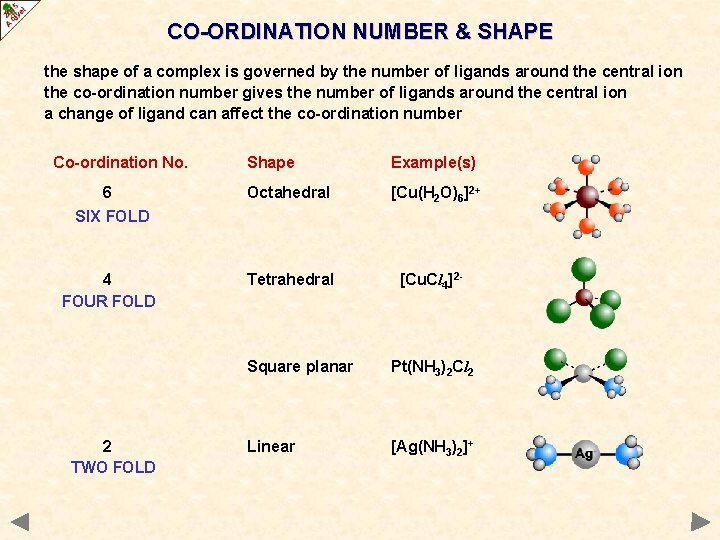
CO-ORDINATION NUMBER & SHAPE the shape of a complex is governed by the number of ligands around the central ion the co-ordination number gives the number of ligands around the central ion a change of ligand can affect the co-ordination number Co-ordination No. Shape Example(s) 6 SIX FOLD Octahedral [Cu(H 2 O)6]2+ 4 FOUR FOLD Tetrahedral [Cu. Cl 4]2 - 2 TWO FOLD Square planar Pt(NH 3)2 Cl 2 Linear [Ag(NH 3)2]+
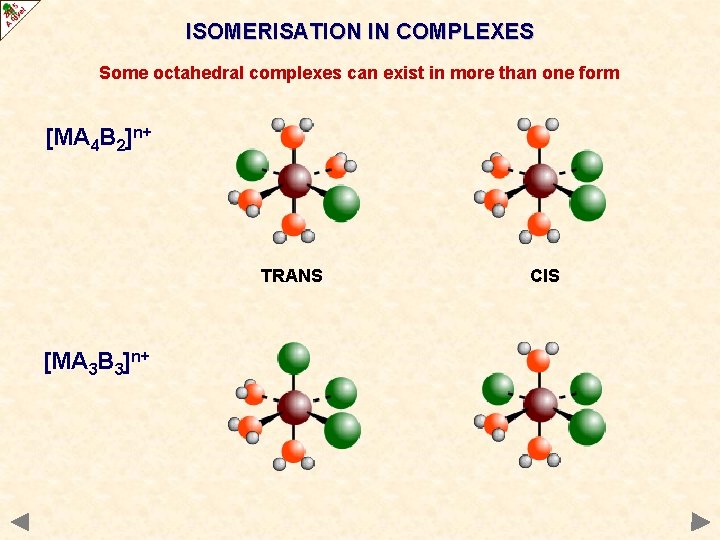
ISOMERISATION IN COMPLEXES Some octahedral complexes can exist in more than one form [MA 4 B 2]n+ TRANS [MA 3 B 3]n+ CIS
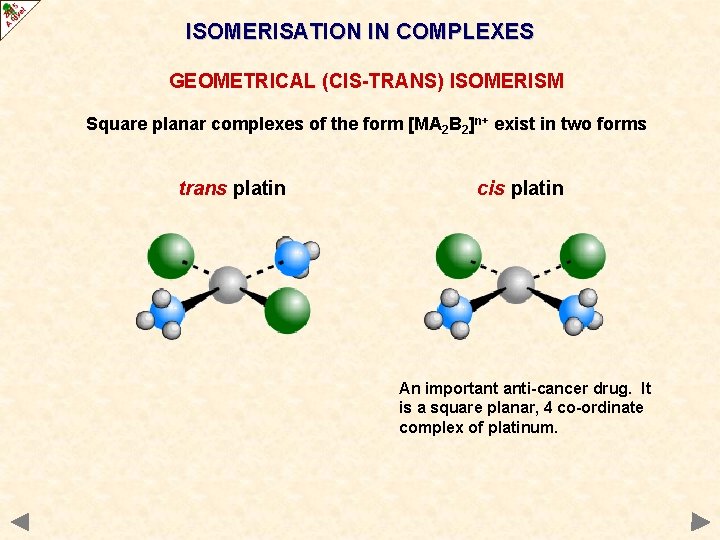
ISOMERISATION IN COMPLEXES GEOMETRICAL (CIS-TRANS) ISOMERISM Square planar complexes of the form [MA 2 B 2]n+ exist in two forms trans platin cis platin An important anti-cancer drug. It is a square planar, 4 co-ordinate complex of platinum.
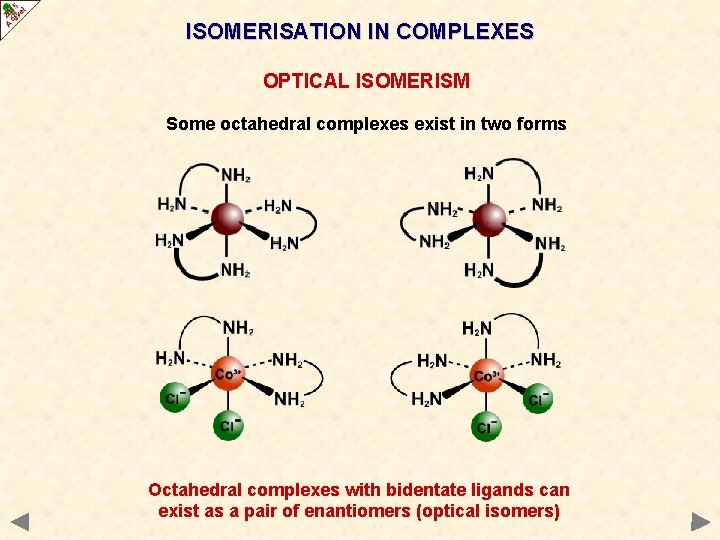
ISOMERISATION IN COMPLEXES OPTICAL ISOMERISM Some octahedral complexes exist in two forms Octahedral complexes with bidentate ligands can exist as a pair of enantiomers (optical isomers)
![ISOMERISATION IN COMPLEXES OPTICAL ISOMERISM AND GEOMETRICAL ISOMERSIM The complex ion Coen2 Cl 2 ISOMERISATION IN COMPLEXES OPTICAL ISOMERISM AND GEOMETRICAL ISOMERSIM The complex ion [Co(en)2 Cl 2]+](https://slidetodoc.com/presentation_image_h/361e6347eb1946d07d7ff4d7d6d4a521/image-41.jpg)
ISOMERISATION IN COMPLEXES OPTICAL ISOMERISM AND GEOMETRICAL ISOMERSIM The complex ion [Co(en)2 Cl 2]+ exhibits both types of isomerism OPTICAL ISOMERISM
![ISOMERISATION IN COMPLEXES OPTICAL ISOMERISM AND GEOMETRICAL ISOMERSIM The complex ion Coen2 Cl 2 ISOMERISATION IN COMPLEXES OPTICAL ISOMERISM AND GEOMETRICAL ISOMERSIM The complex ion [Co(en)2 Cl 2]+](https://slidetodoc.com/presentation_image_h/361e6347eb1946d07d7ff4d7d6d4a521/image-42.jpg)
ISOMERISATION IN COMPLEXES OPTICAL ISOMERISM AND GEOMETRICAL ISOMERSIM The complex ion [Co(en)2 Cl 2]+ exhibits both types of isomerism OPTICAL ISOMERISM GEOMETRICAL ISOMERISM CIS TRANS
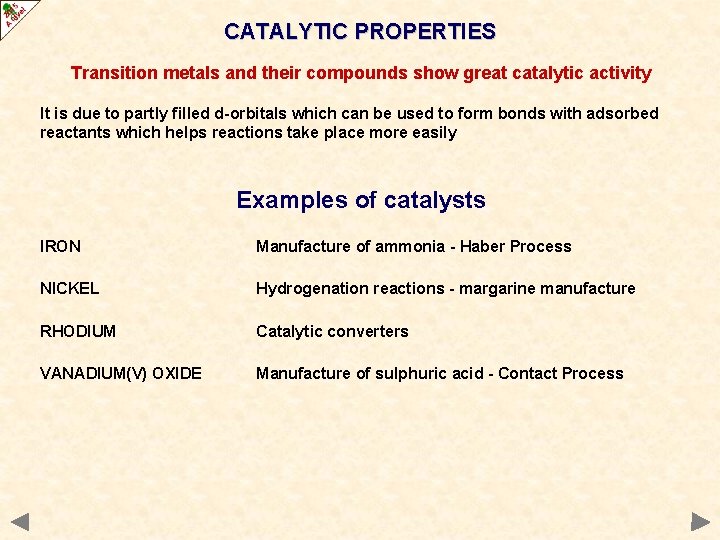
CATALYTIC PROPERTIES Transition metals and their compounds show great catalytic activity It is due to partly filled d-orbitals which can be used to form bonds with adsorbed reactants which helps reactions take place more easily Examples of catalysts IRON Manufacture of ammonia - Haber Process NICKEL Hydrogenation reactions - margarine manufacture RHODIUM Catalytic converters VANADIUM(V) OXIDE Manufacture of sulphuric acid - Contact Process
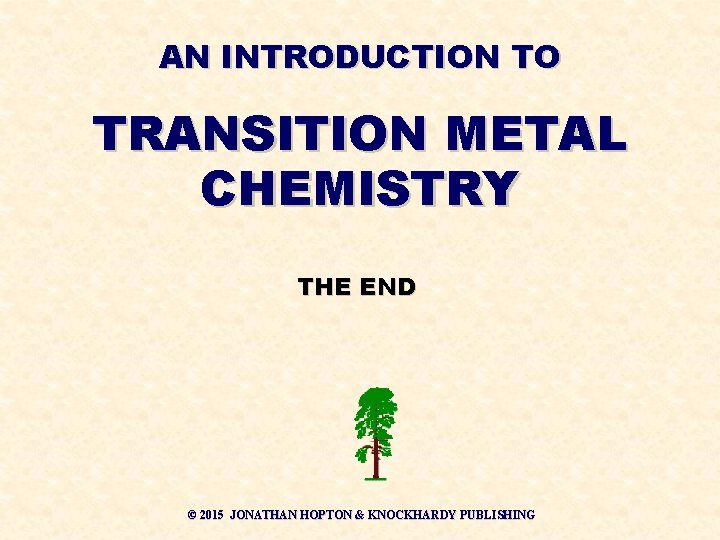
AN INTRODUCTION TO TRANSITION METAL CHEMISTRY THE END © 2015 JONATHAN HOPTON & KNOCKHARDY PUBLISHING
Order of increasing first ionization energy
Lattice energy trend
Thermal decomposition of group 1 nitrates
Order of increasing first ionization energy
Increasing lattice energy trend
Non-metal halogen family atomic mass 35
Transition metal ion colours
Spectrochemical series
Transition metal ion colours
Racah parameter and nephelauxetic effect
Orgel diagram is used for
A transition metal used in incandescent light bulbs
Diagram states of matter
Metals vs nonmetals properties
Acidity trends periodic table
Compare the properties of metals and nonmetals
Pmos nwell
Metals
Periodic table pure substances
When a metal reacts with a nonmetal the metal will
P
Chemical bond def
Clasificación de los metales y no metales
Examples of non metals
Electrones de valencia de francio
Transition state diagram chemistry
Energy energy transfer and general energy analysis
Energy energy transfer and general energy analysis
Ib chemistry functional groups
Inorganic vs organic chemistry
Energy reaction coordinate diagram
Ionization energy of transition metals
Rearranged most stable carbocation is
Edmonton energy transition strategy
Introduction of sheet metal
Sheet metal introduction
Area of sheet metal
Binding energy chemistry
Kinetic energy
Ap chem spontaneity entropy and free energy
Born haber cycle
E=mc2 cda
Free energy in chemistry
White tiger population graph
How to arrange elements in increasing atomic size