Spectroscopic and related techniques in surface science for
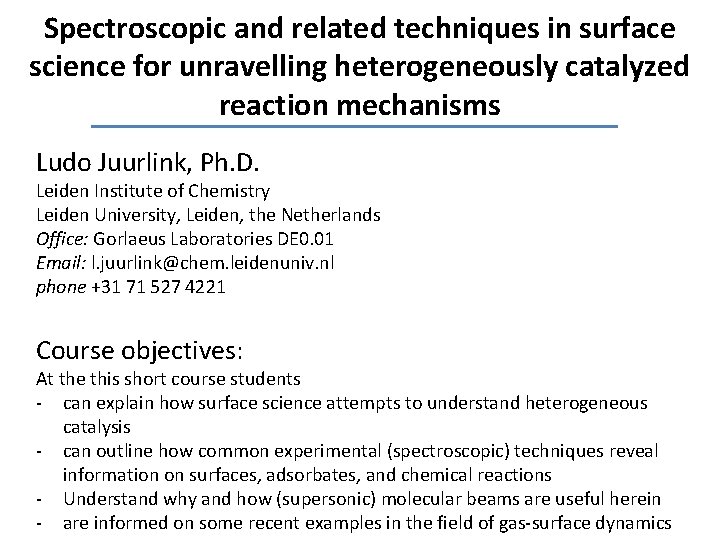
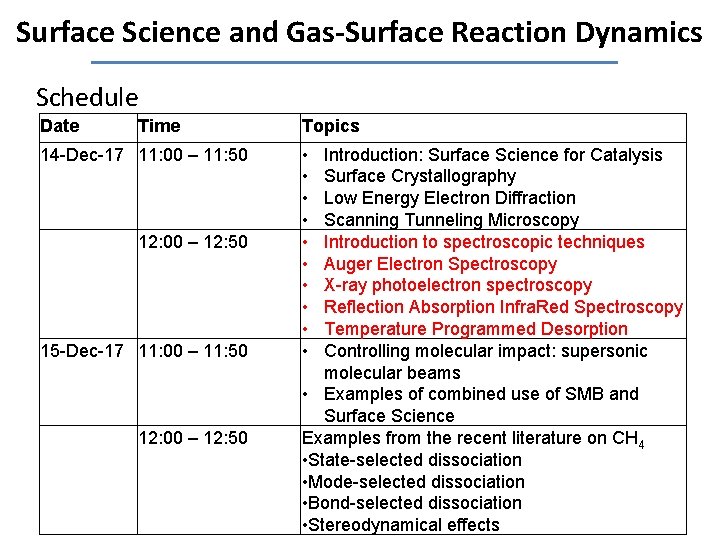
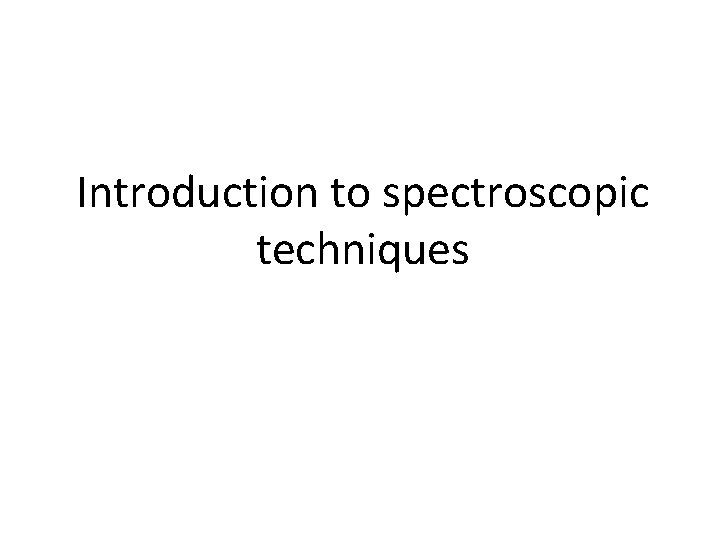
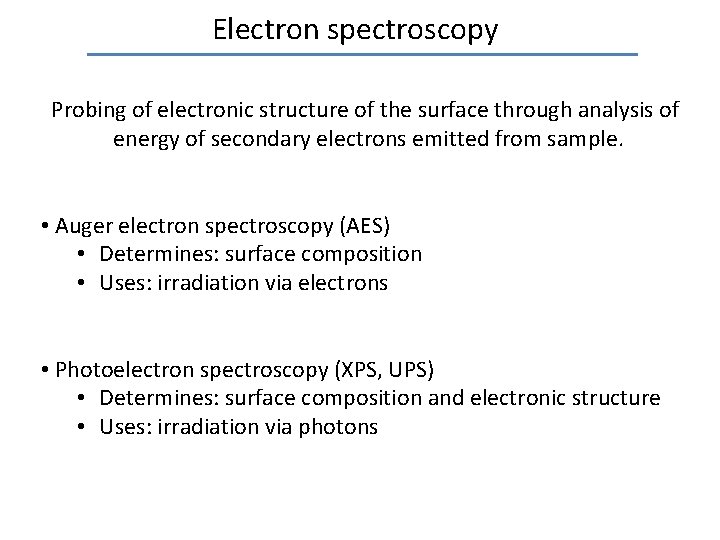
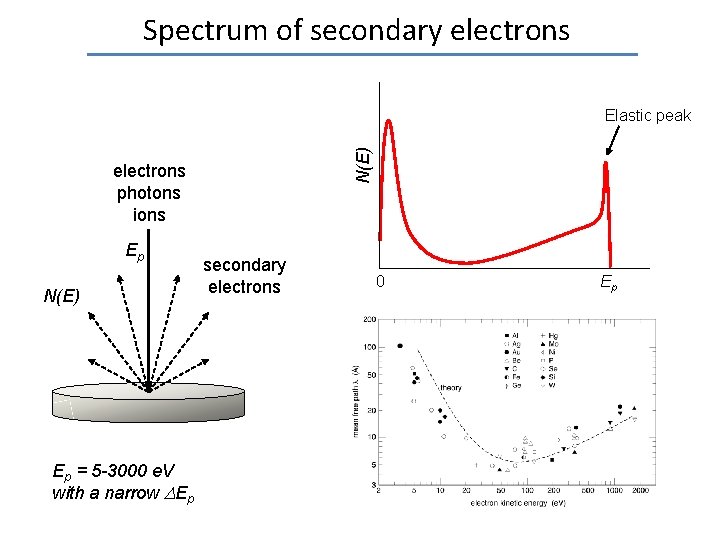
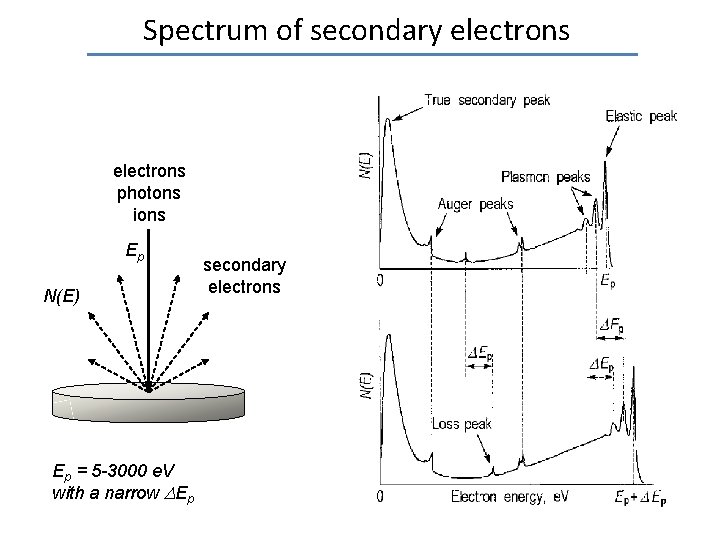
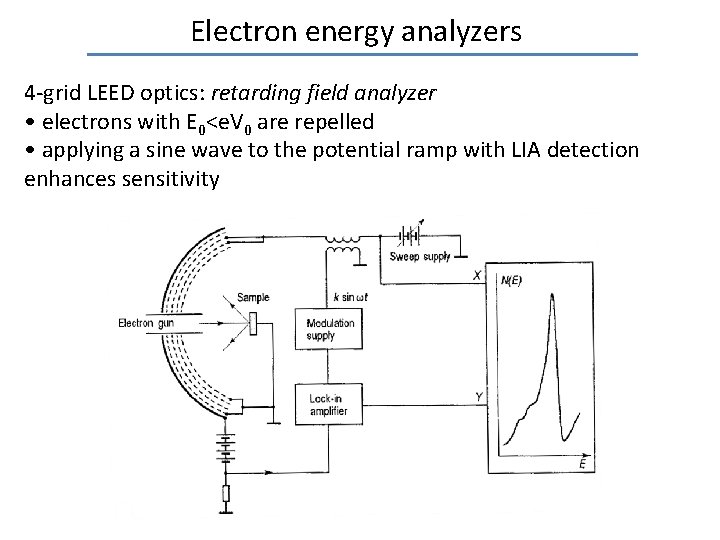
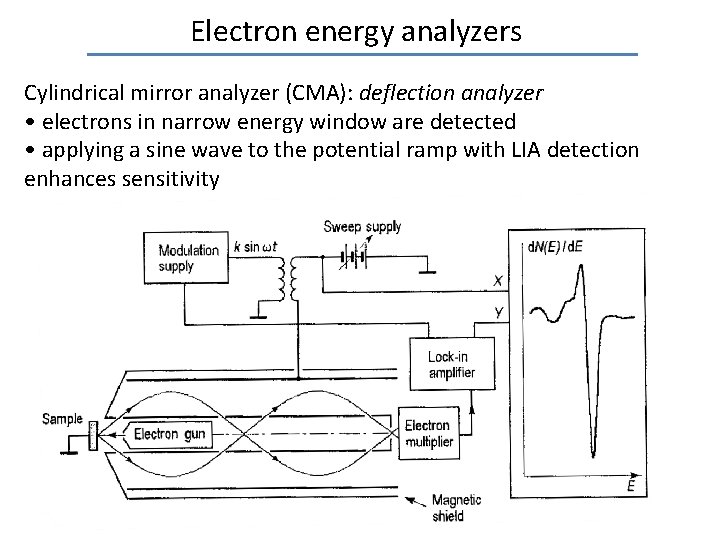
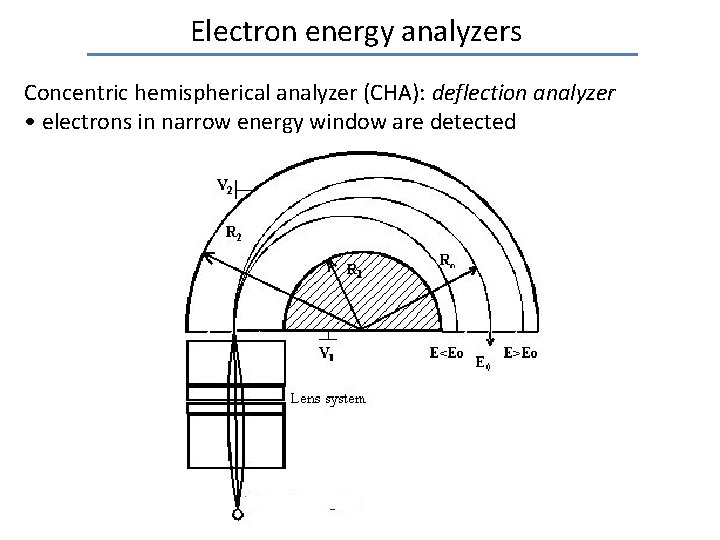
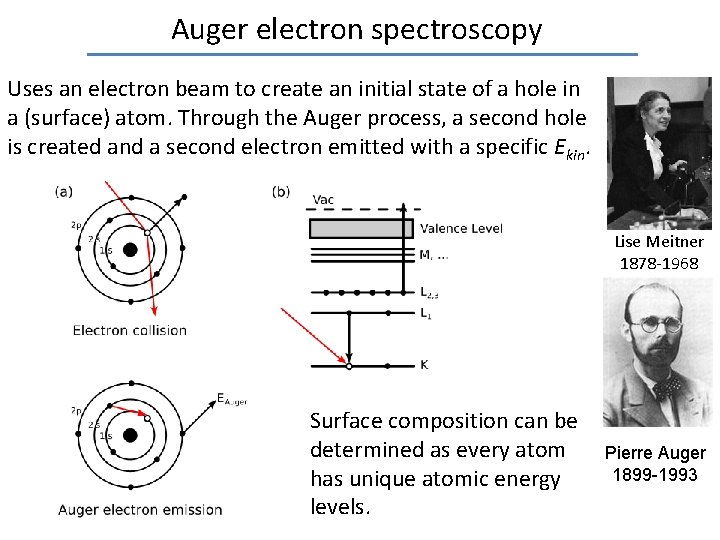
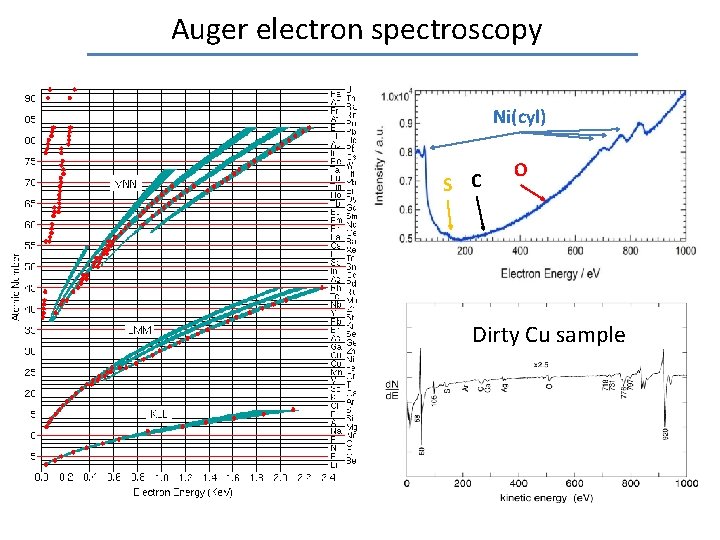
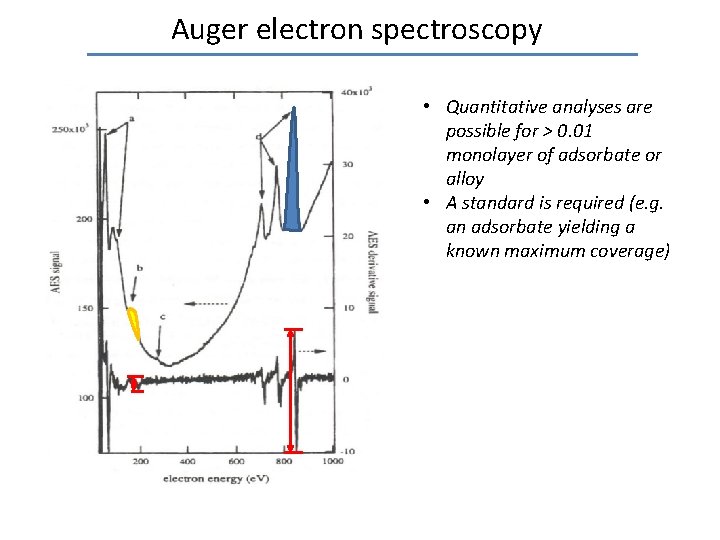
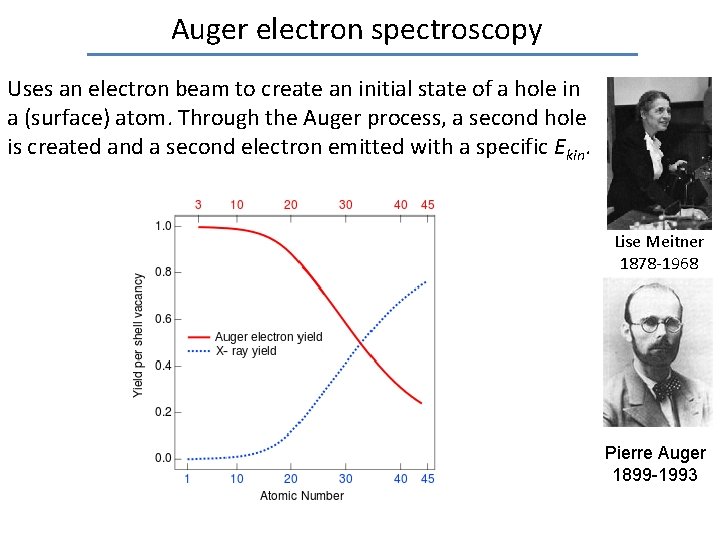
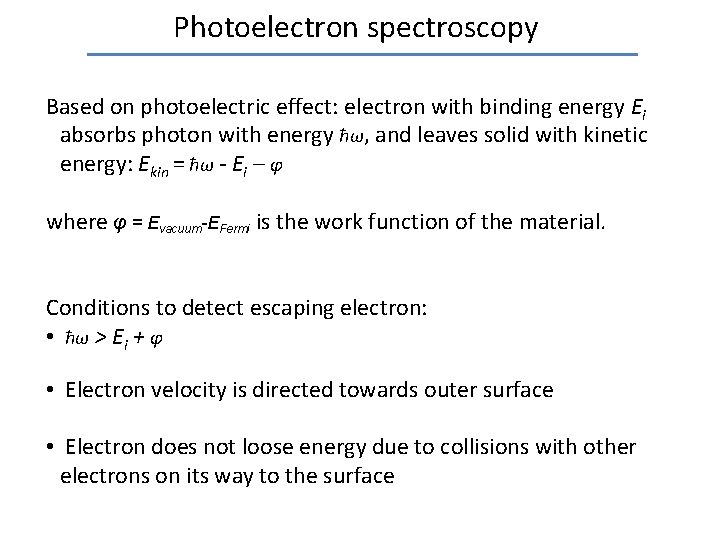
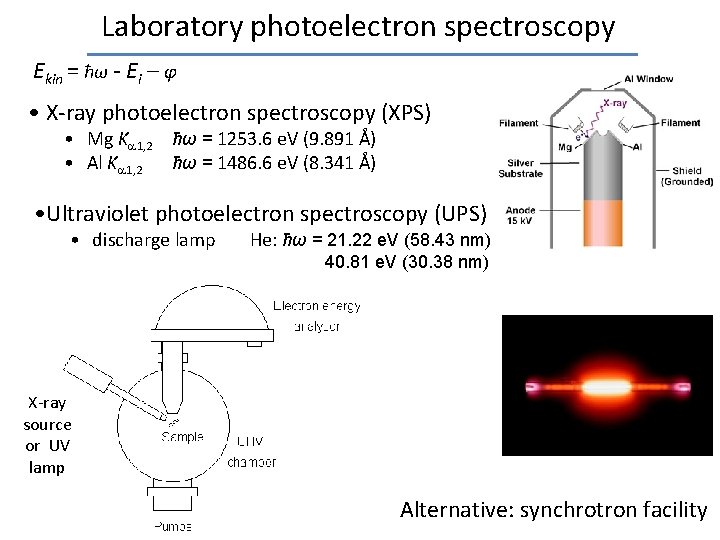
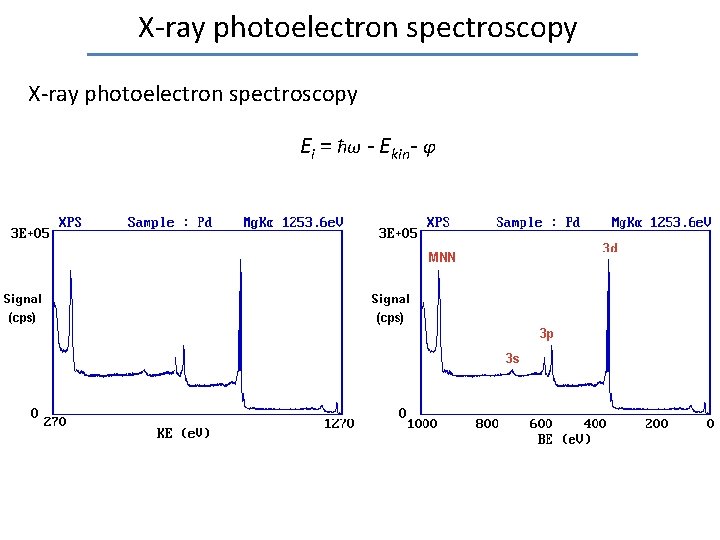
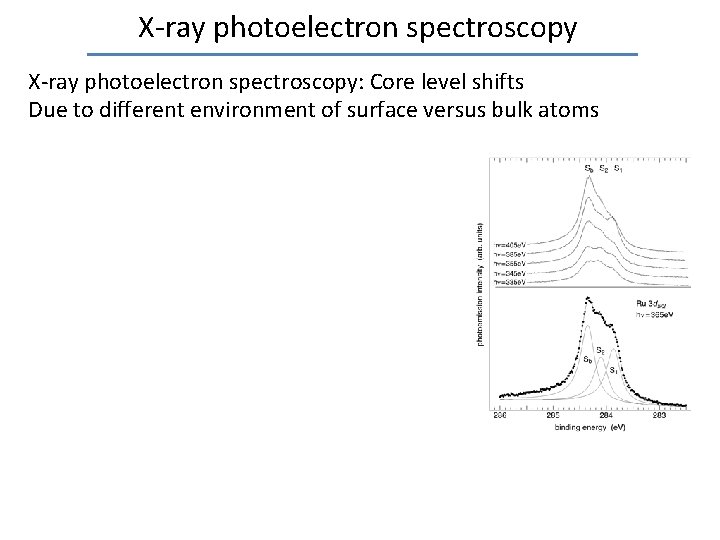
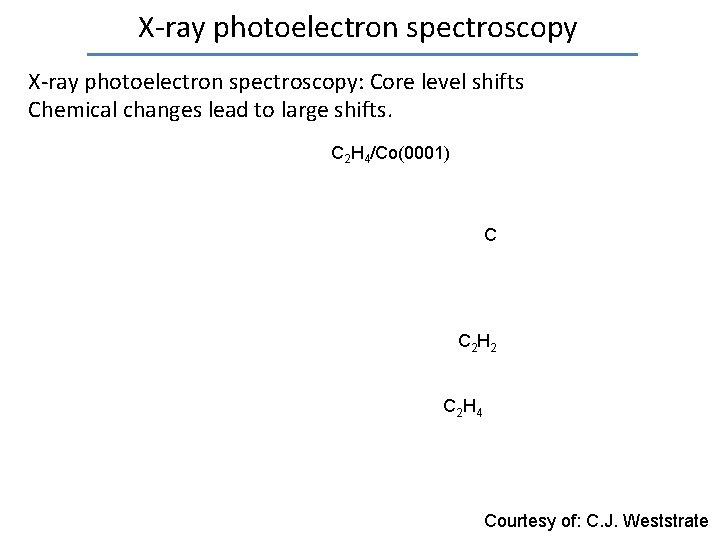
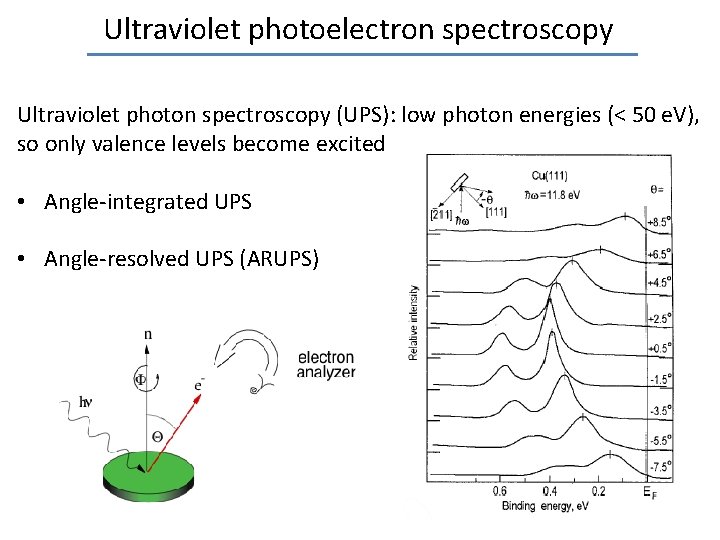
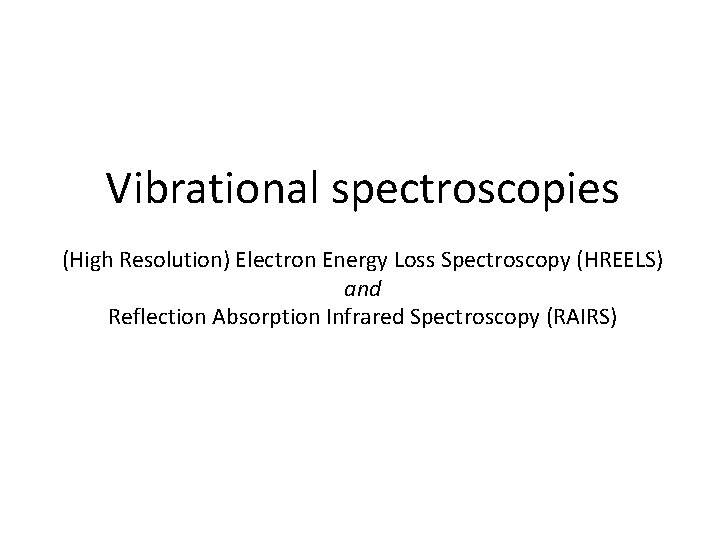
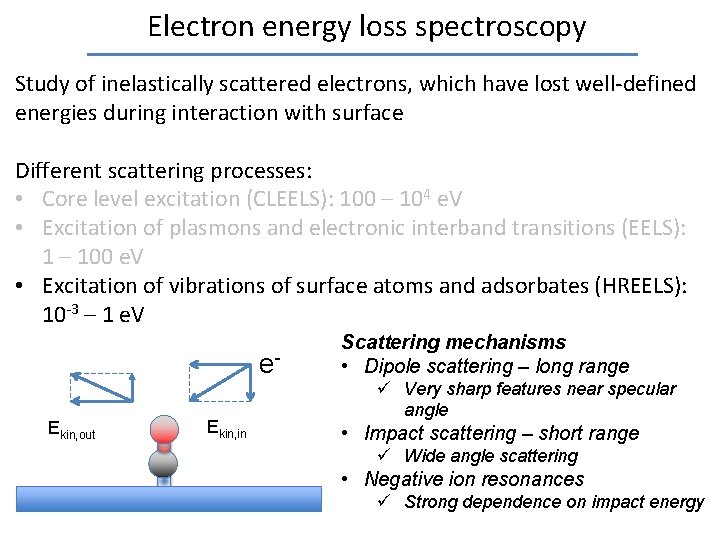
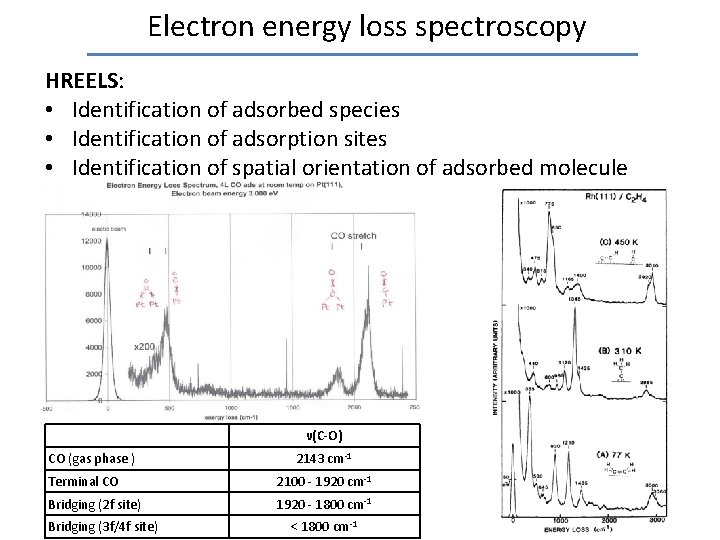
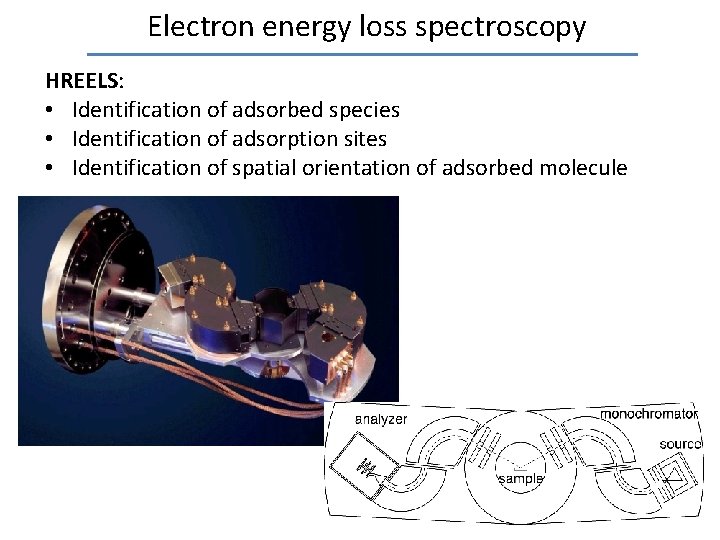
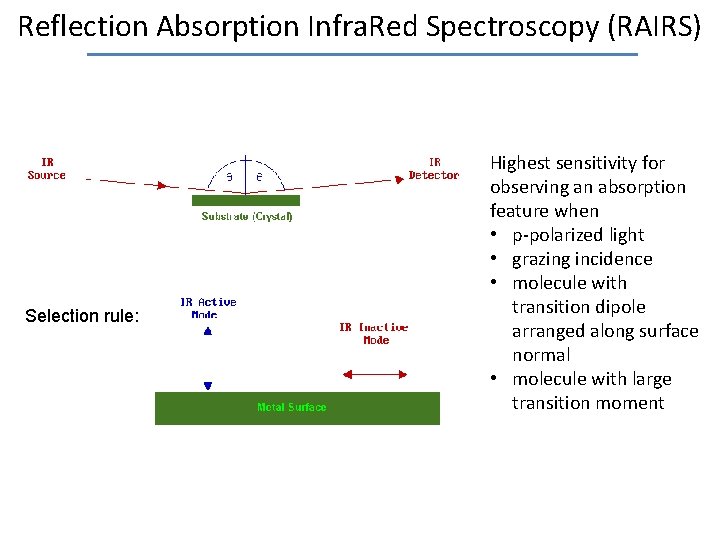
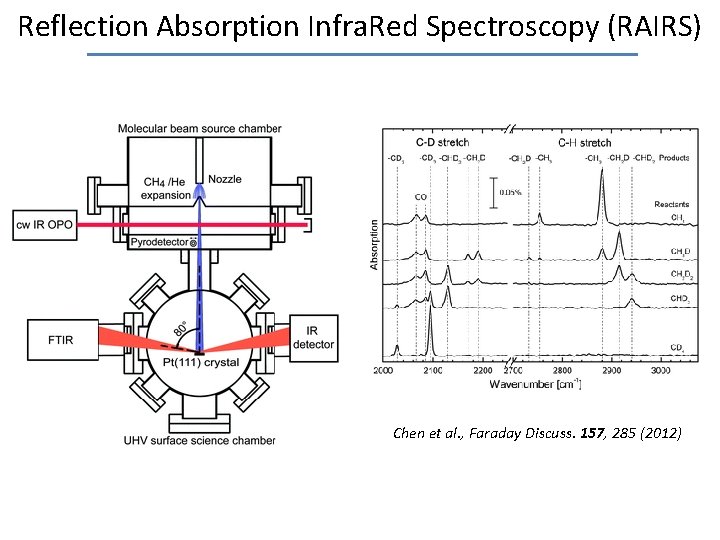
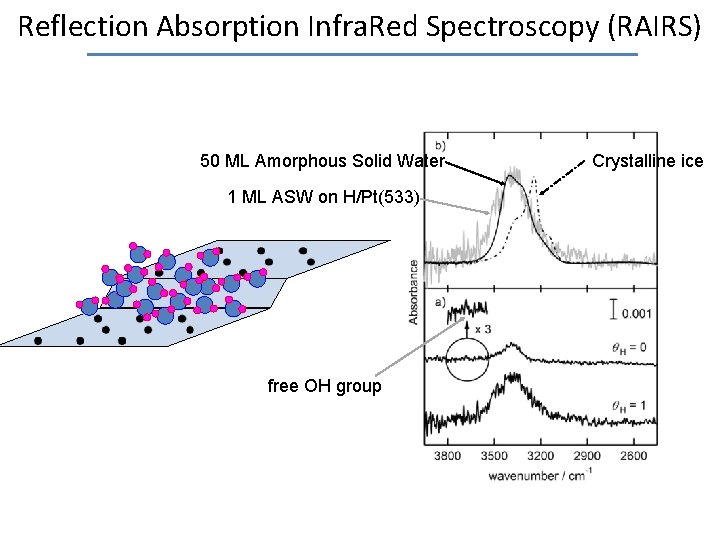
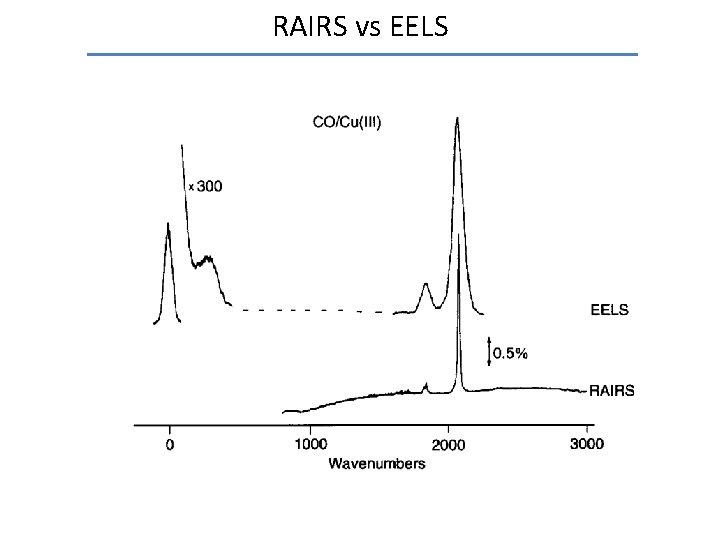
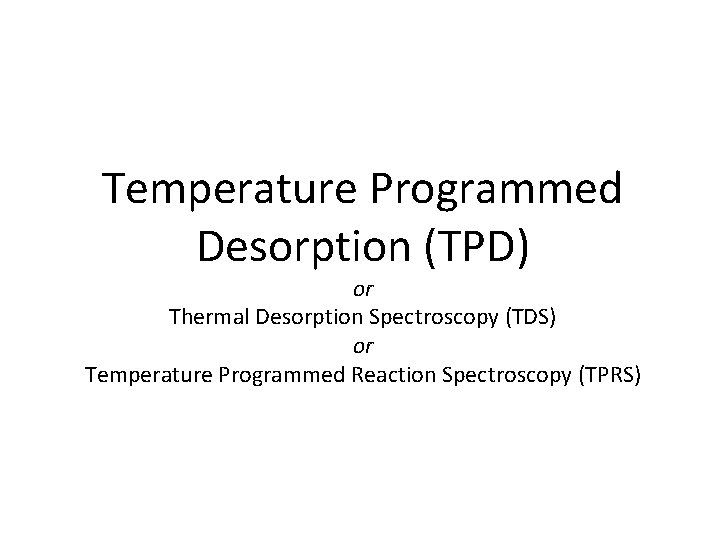
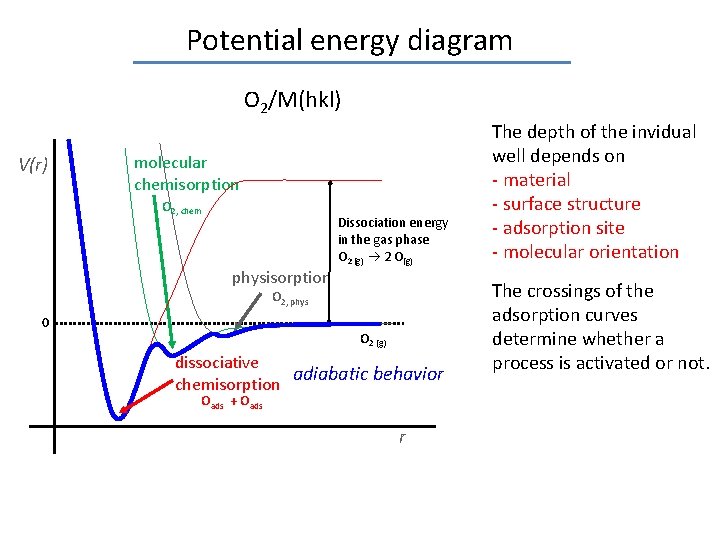
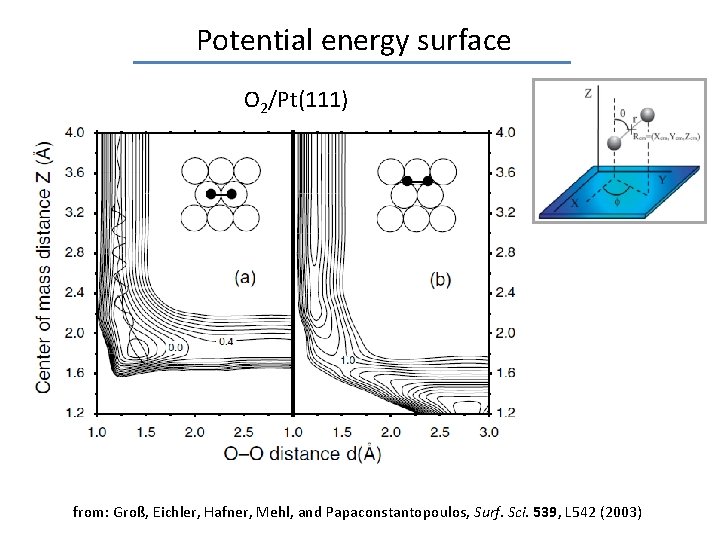
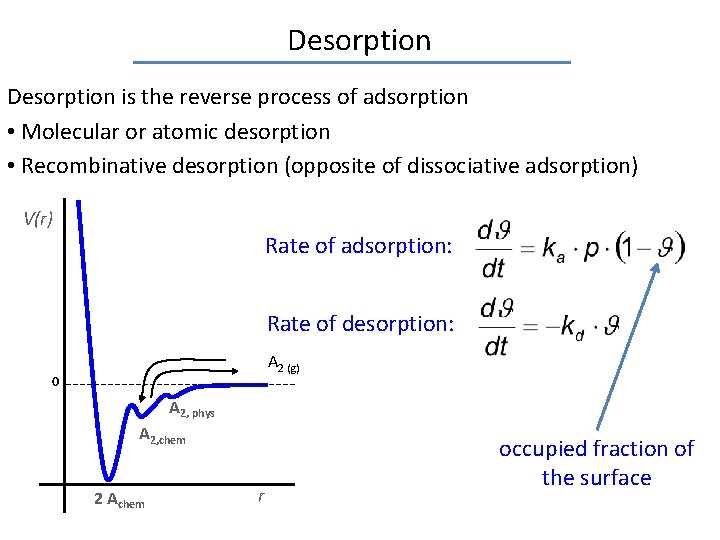
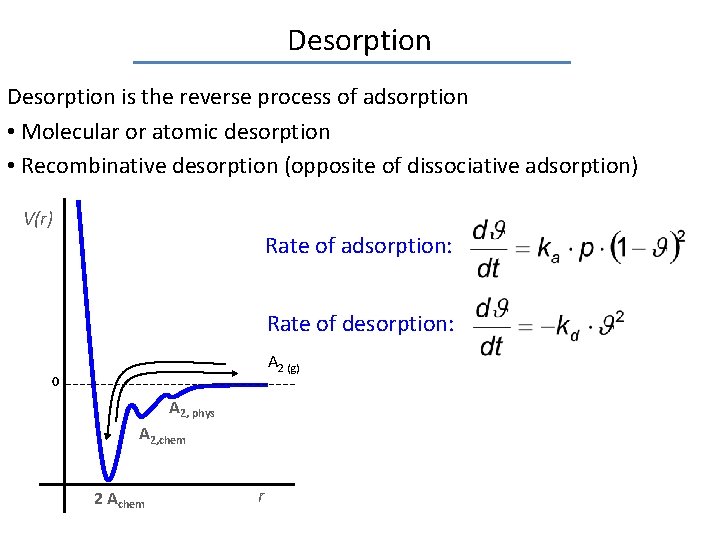
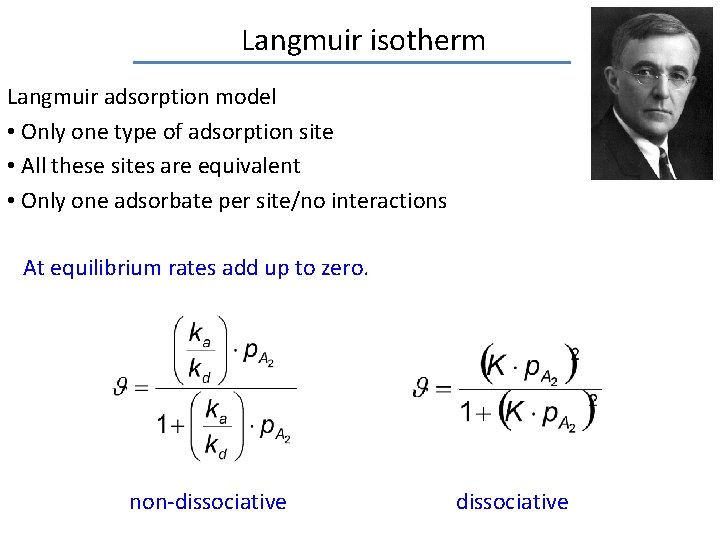
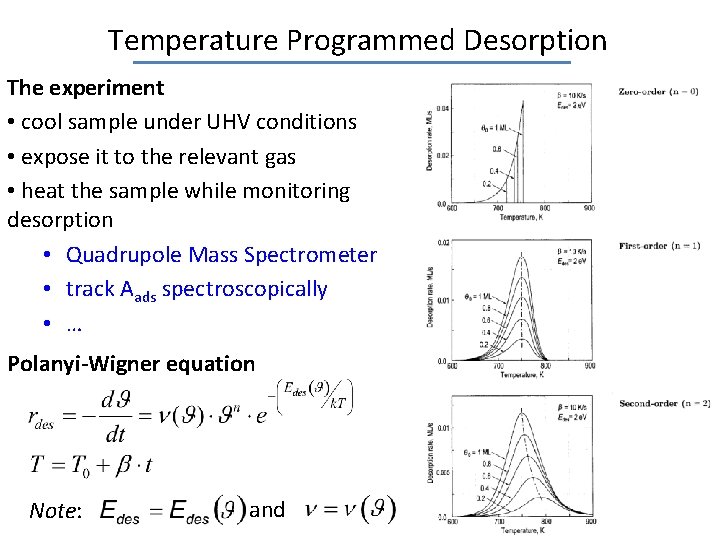
![Example H 2 O interaction with Pt[n(111)x(100)] Example H 2 O interaction with Pt[n(111)x(100)]](https://slidetodoc.com/presentation_image_h/98256ba861f6f16582625ea403be9ccc/image-35.jpg)
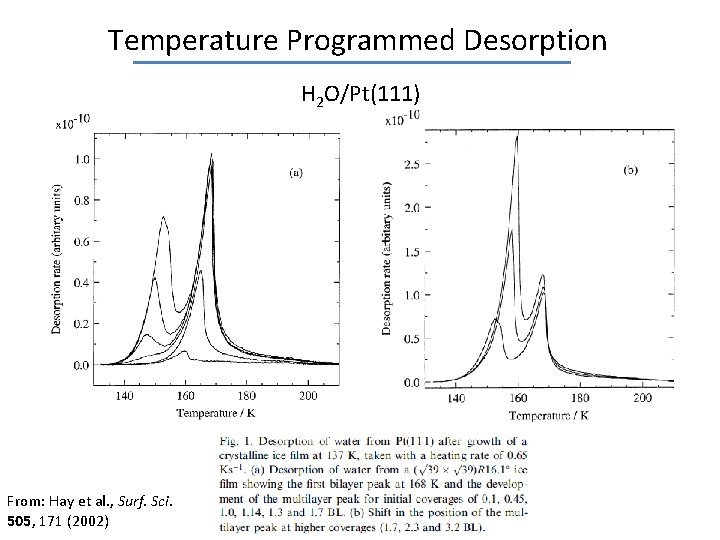
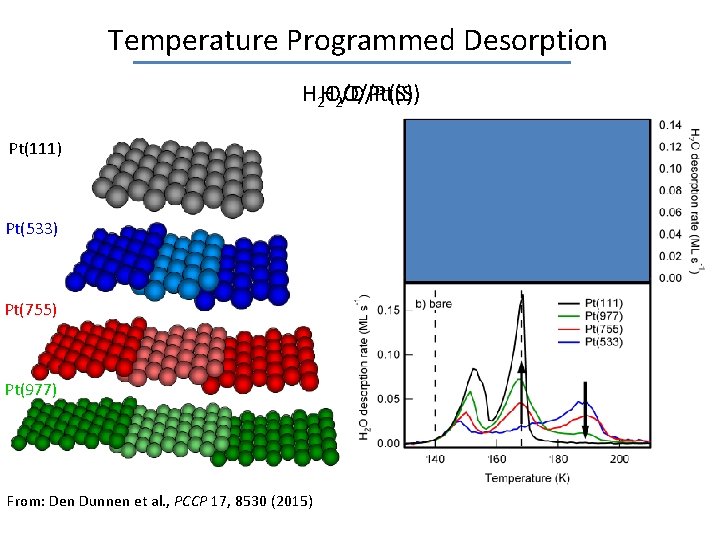
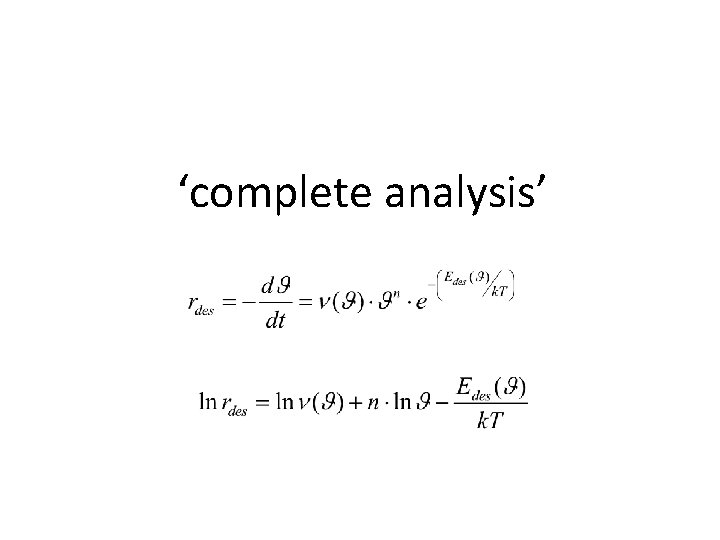
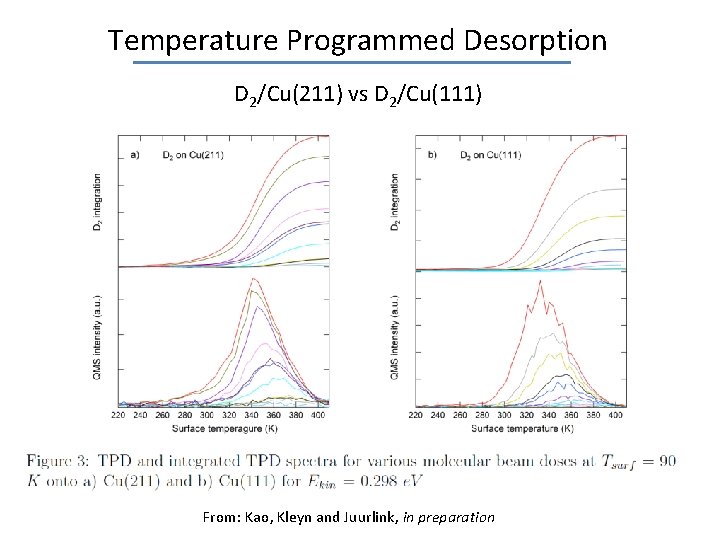
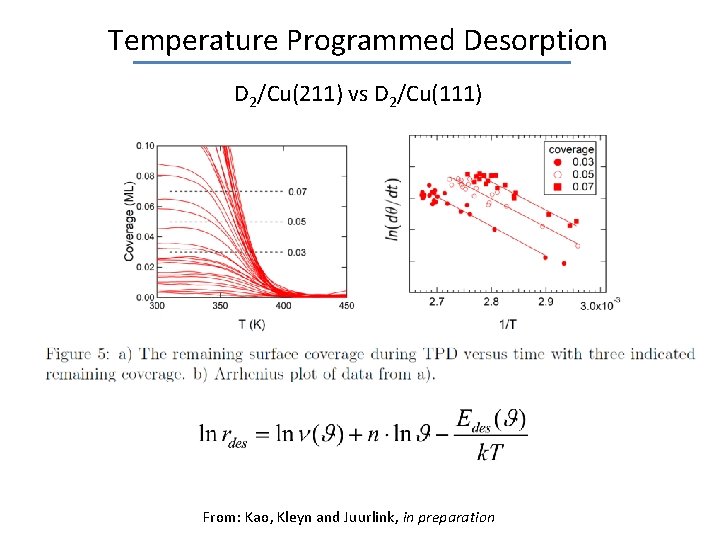
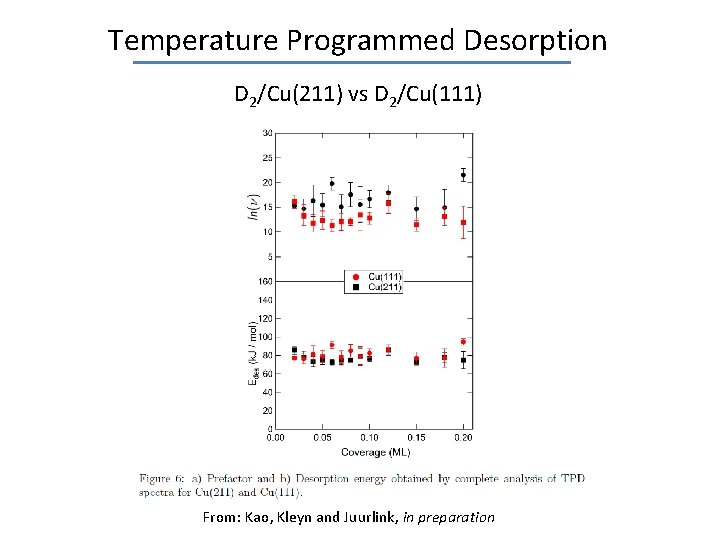
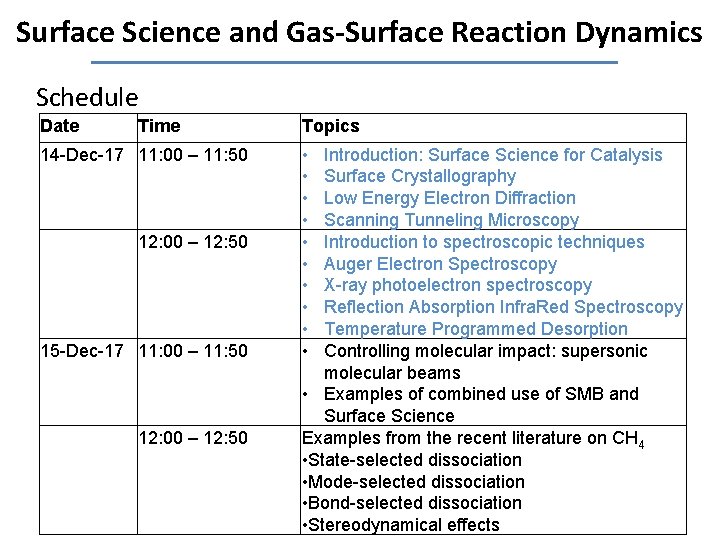
- Slides: 42
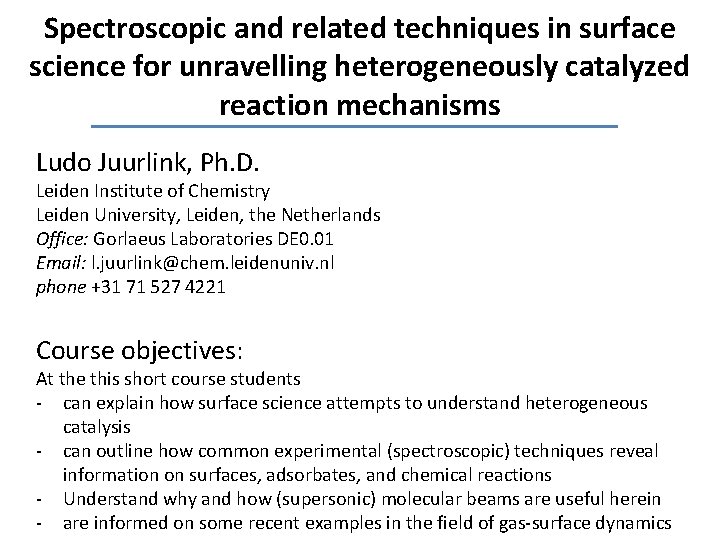
Spectroscopic and related techniques in surface science for unravelling heterogeneously catalyzed reaction mechanisms Ludo Juurlink, Ph. D. Leiden Institute of Chemistry Leiden University, Leiden, the Netherlands Office: Gorlaeus Laboratories DE 0. 01 Email: l. juurlink@chem. leidenuniv. nl phone +31 71 527 4221 Course objectives: At the this short course students - can explain how surface science attempts to understand heterogeneous catalysis - can outline how common experimental (spectroscopic) techniques reveal information on surfaces, adsorbates, and chemical reactions - Understand why and how (supersonic) molecular beams are useful herein - are informed on some recent examples in the field of gas-surface dynamics
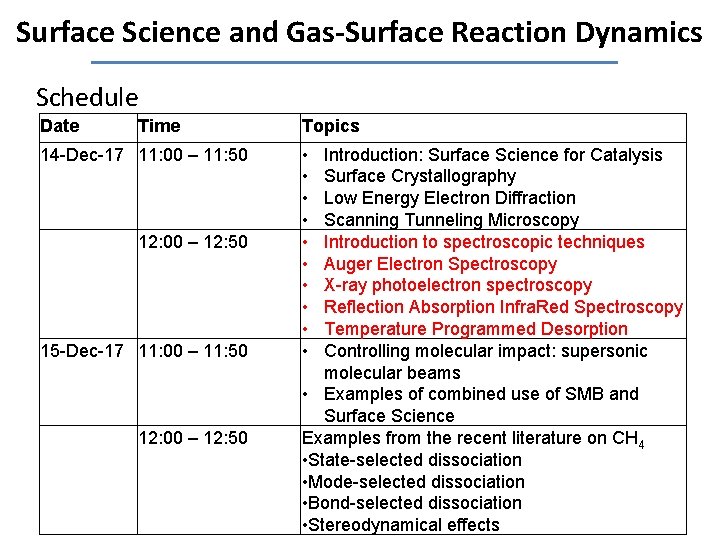
Surface Science and Gas-Surface Reaction Dynamics Schedule Date Time 14 -Dec-17 11: 00 – 11: 50 12: 00 – 12: 50 15 -Dec-17 11: 00 – 11: 50 12: 00 – 12: 50 Topics • • • Introduction: Surface Science for Catalysis Surface Crystallography Low Energy Electron Diffraction Scanning Tunneling Microscopy Introduction to spectroscopic techniques Auger Electron Spectroscopy X-ray photoelectron spectroscopy Reflection Absorption Infra. Red Spectroscopy Temperature Programmed Desorption Controlling molecular impact: supersonic molecular beams • Examples of combined use of SMB and Surface Science Examples from the recent literature on CH 4 • State-selected dissociation • Mode-selected dissociation • Bond-selected dissociation • Stereodynamical effects
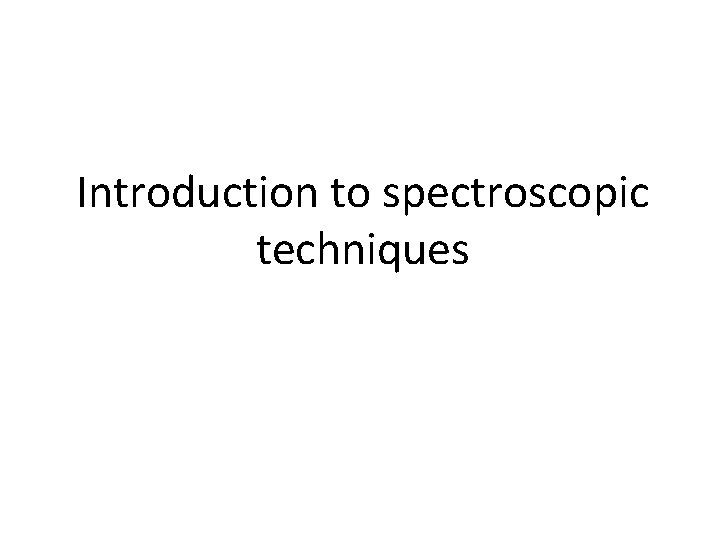
Introduction to spectroscopic techniques
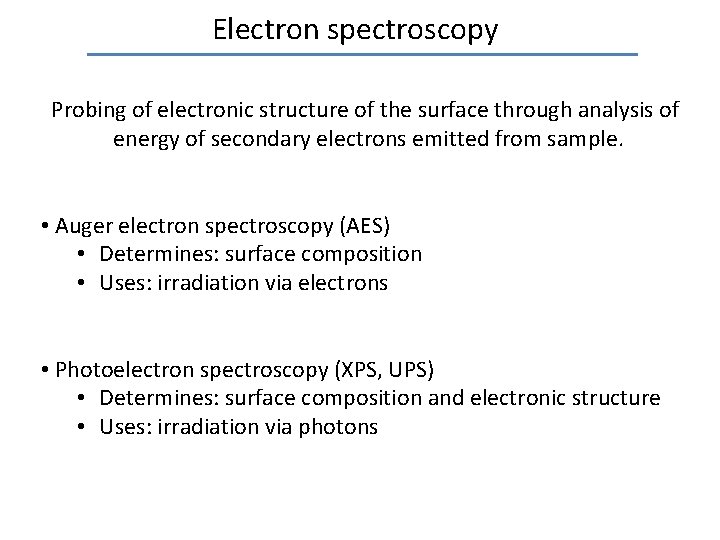
Electron spectroscopy Probing of electronic structure of the surface through analysis of energy of secondary electrons emitted from sample. • Auger electron spectroscopy (AES) • Determines: surface composition • Uses: irradiation via electrons • Photoelectron spectroscopy (XPS, UPS) • Determines: surface composition and electronic structure • Uses: irradiation via photons
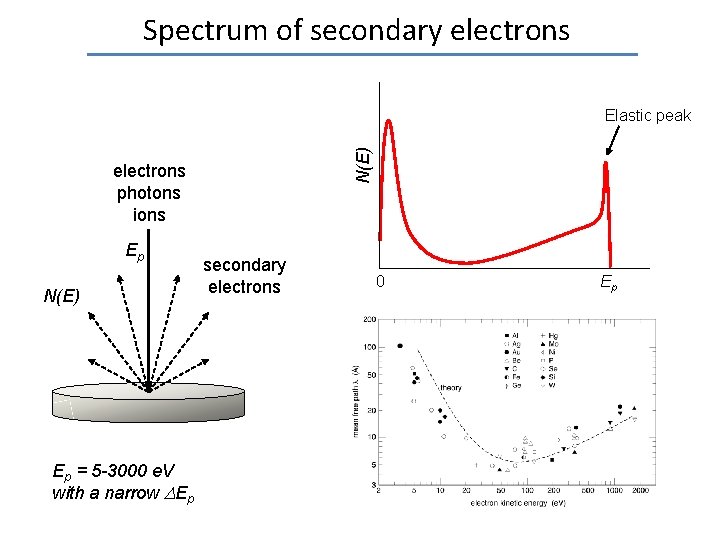
Spectrum of secondary electrons N(E) Elastic peak electrons photons ions Ep N(E) Ep = 5 -3000 e. V with a narrow Ep secondary electrons 0 Ep
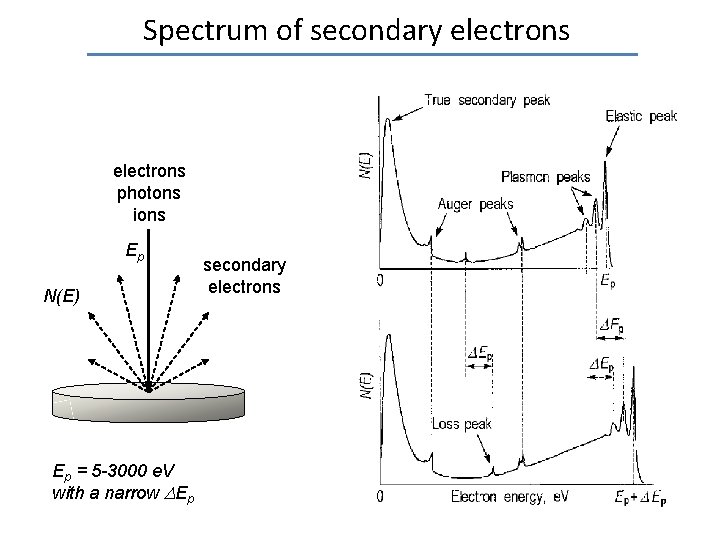
Spectrum of secondary electrons photons ions Ep N(E) Ep = 5 -3000 e. V with a narrow Ep secondary electrons
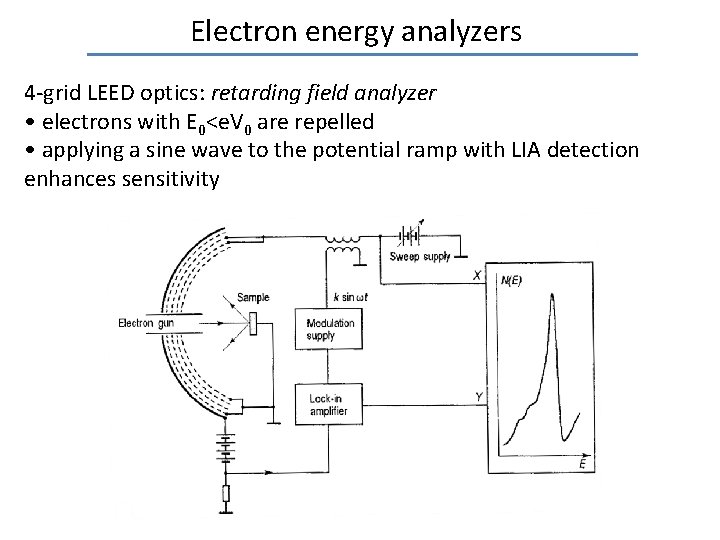
Electron energy analyzers 4 -grid LEED optics: retarding field analyzer • electrons with E 0<e. V 0 are repelled • applying a sine wave to the potential ramp with LIA detection enhances sensitivity
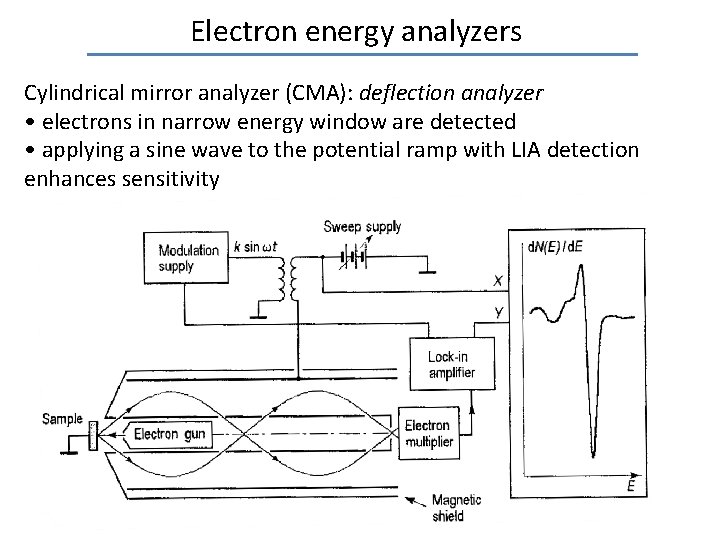
Electron energy analyzers Cylindrical mirror analyzer (CMA): deflection analyzer • electrons in narrow energy window are detected • applying a sine wave to the potential ramp with LIA detection enhances sensitivity
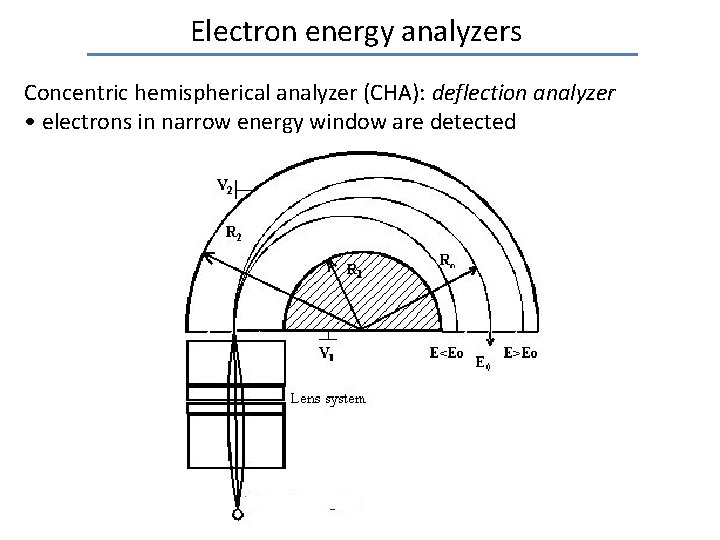
Electron energy analyzers Concentric hemispherical analyzer (CHA): deflection analyzer • electrons in narrow energy window are detected
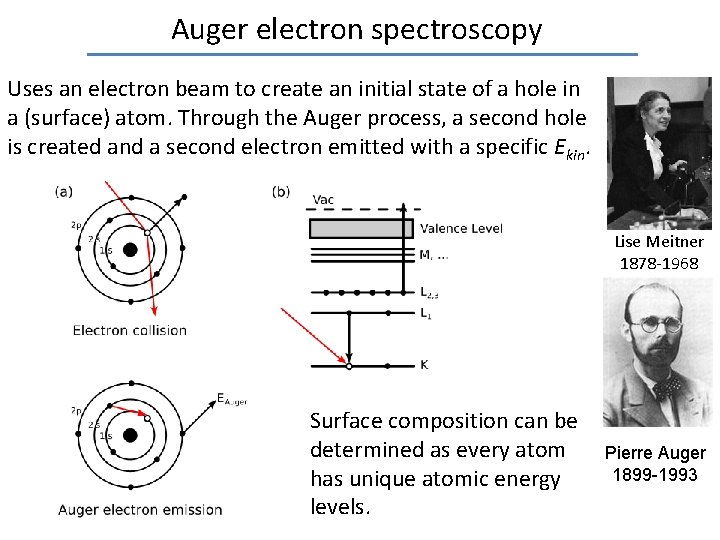
Auger electron spectroscopy Uses an electron beam to create an initial state of a hole in a (surface) atom. Through the Auger process, a second hole is created and a second electron emitted with a specific Ekin. Lise Meitner 1878 -1968 Surface composition can be determined as every atom has unique atomic energy levels. Pierre Auger 1899 -1993
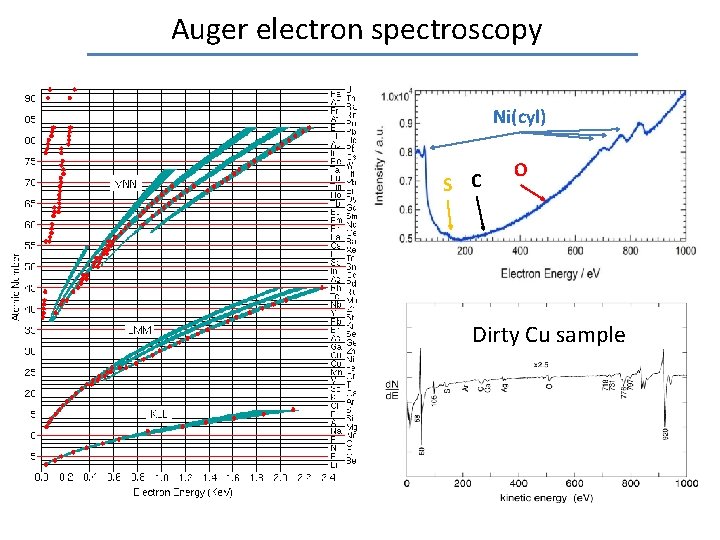
Auger electron spectroscopy Ni(cyl) S C O Dirty Cu sample
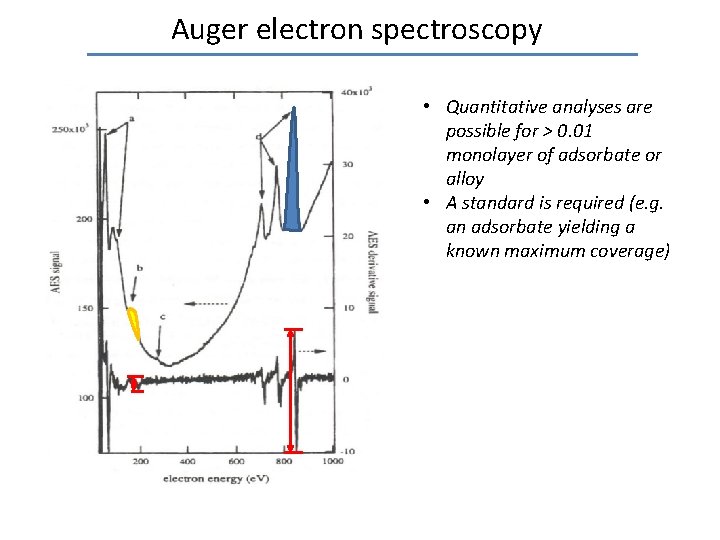
Auger electron spectroscopy • Quantitative analyses are possible for > 0. 01 monolayer of adsorbate or alloy • A standard is required (e. g. an adsorbate yielding a known maximum coverage)
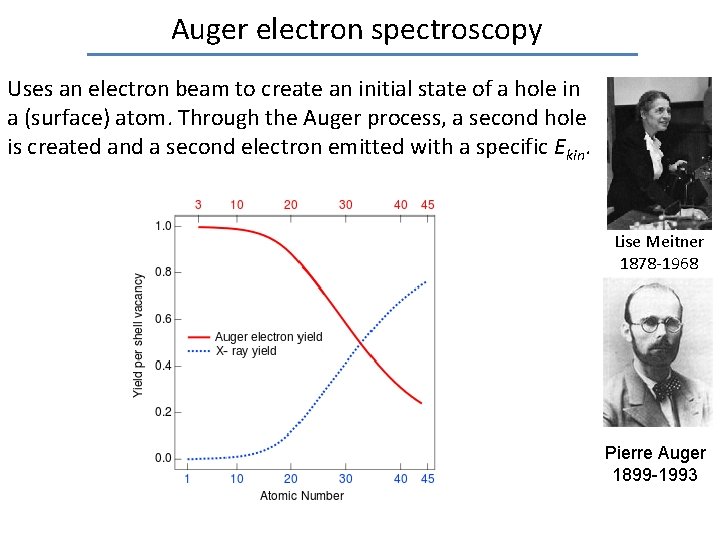
Auger electron spectroscopy Uses an electron beam to create an initial state of a hole in a (surface) atom. Through the Auger process, a second hole is created and a second electron emitted with a specific Ekin. Lise Meitner 1878 -1968 Pierre Auger 1899 -1993
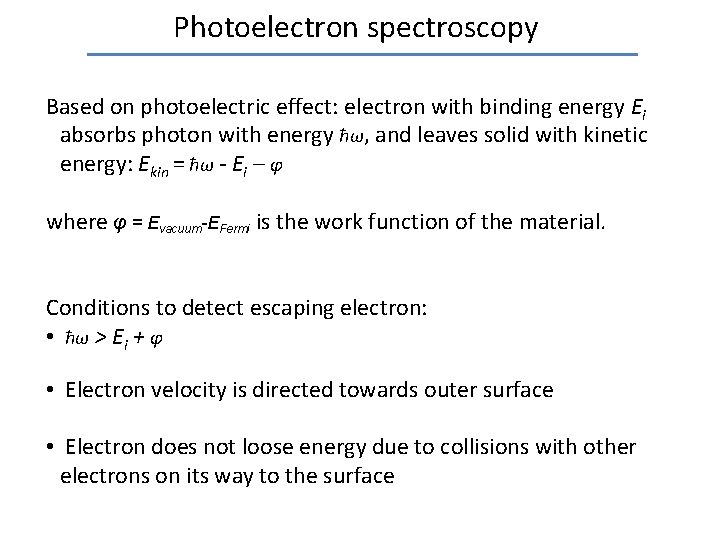
Photoelectron spectroscopy Based on photoelectric effect: electron with binding energy Ei absorbs photon with energy ħω, and leaves solid with kinetic energy: Ekin = ħω - Ei – φ where φ = Evacuum-EFermi is the work function of the material. Conditions to detect escaping electron: • ħω > Ei + φ • Electron velocity is directed towards outer surface • Electron does not loose energy due to collisions with other electrons on its way to the surface
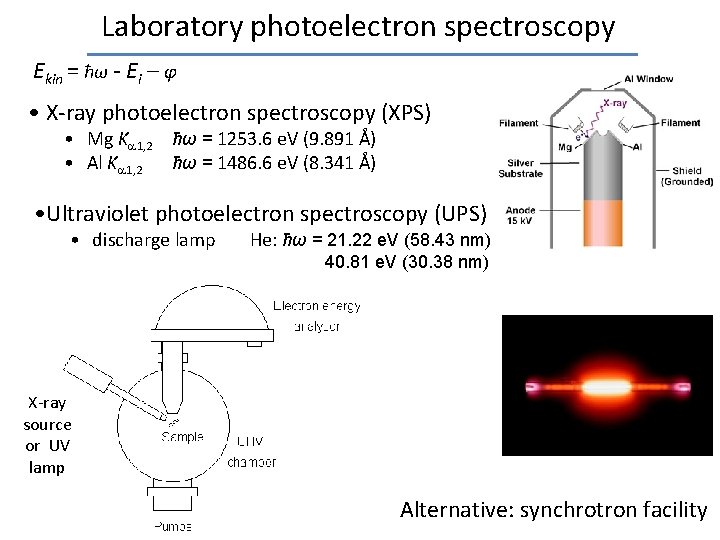
Laboratory photoelectron spectroscopy Ekin = ħω - Ei – φ • X-ray photoelectron spectroscopy (XPS) • Mg K 1, 2 ħω = 1253. 6 e. V (9. 891 Å) • Al K 1, 2 ħω = 1486. 6 e. V (8. 341 Å) • Ultraviolet photoelectron spectroscopy (UPS) • discharge lamp He: ħω = 21. 22 e. V (58. 43 nm) 40. 81 e. V (30. 38 nm) X-ray source or UV lamp Alternative: synchrotron facility
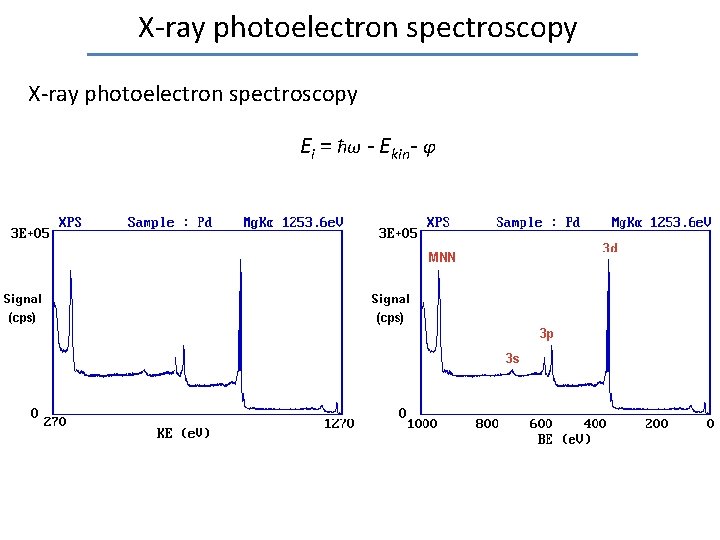
X-ray photoelectron spectroscopy Ei = ħω - Ekin- φ
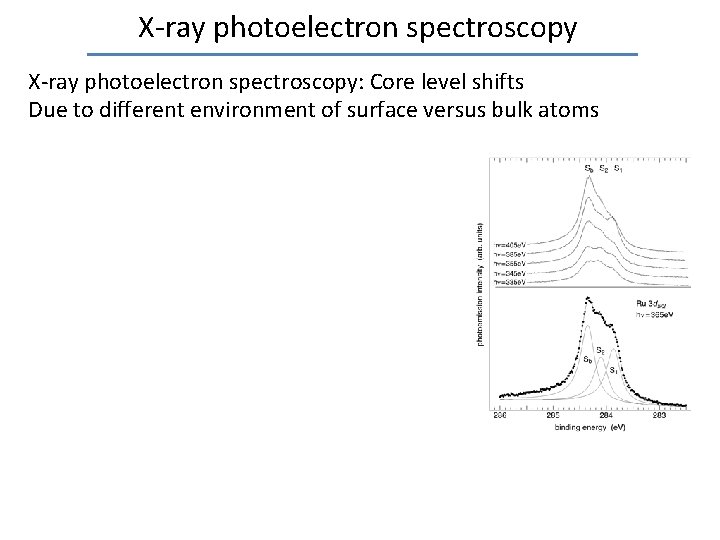
X-ray photoelectron spectroscopy: Core level shifts Due to different environment of surface versus bulk atoms
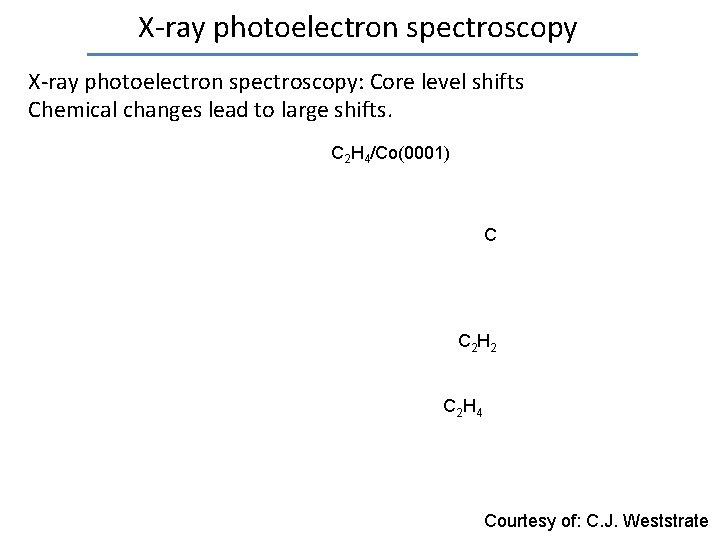
X-ray photoelectron spectroscopy: Core level shifts Chemical changes lead to large shifts. C 2 H 4/Co(0001) C C 2 H 2 C 2 H 4 Courtesy of: C. J. Weststrate
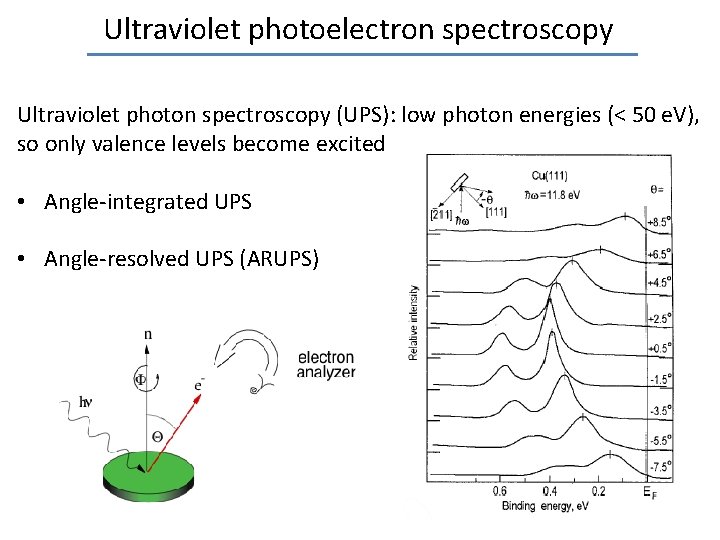
Ultraviolet photoelectron spectroscopy Ultraviolet photon spectroscopy (UPS): low photon energies (< 50 e. V), so only valence levels become excited • Angle-integrated UPS • Angle-resolved UPS (ARUPS)
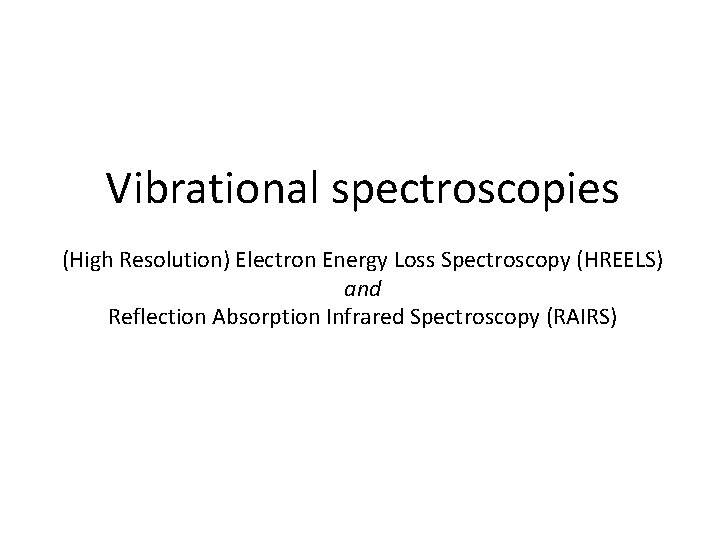
Vibrational spectroscopies (High Resolution) Electron Energy Loss Spectroscopy (HREELS) and Reflection Absorption Infrared Spectroscopy (RAIRS)
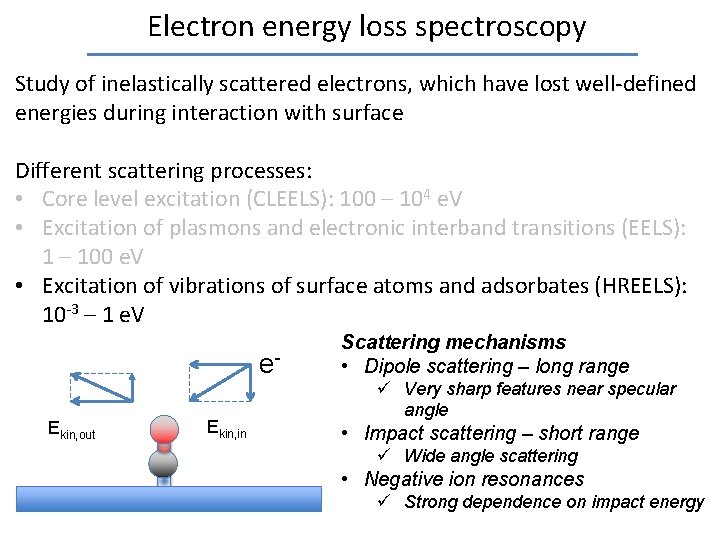
Electron energy loss spectroscopy Study of inelastically scattered electrons, which have lost well-defined energies during interaction with surface Different scattering processes: • Core level excitation (CLEELS): 100 – 104 e. V • Excitation of plasmons and electronic interband transitions (EELS): 1 – 100 e. V • Excitation of vibrations of surface atoms and adsorbates (HREELS): 10 -3 – 1 e. V e. Ekin, out Ekin, in Scattering mechanisms • Dipole scattering – long range ü Very sharp features near specular angle • Impact scattering – short range ü Wide angle scattering • Negative ion resonances ü Strong dependence on impact energy
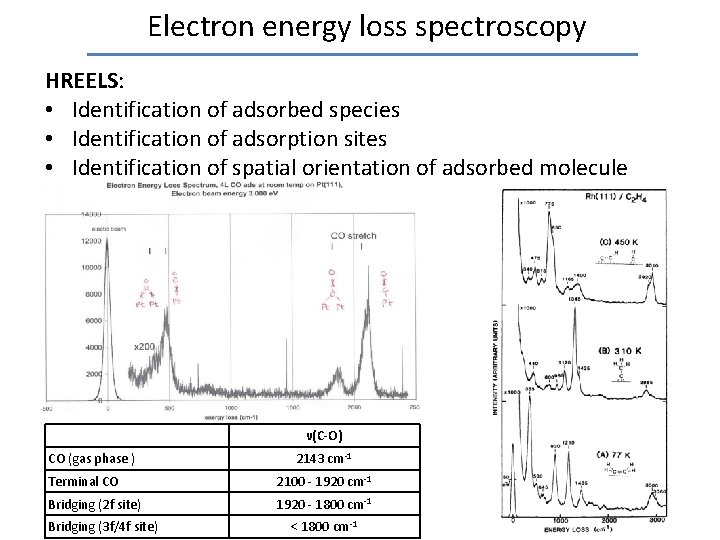
Electron energy loss spectroscopy HREELS: • Identification of adsorbed species • Identification of adsorption sites • Identification of spatial orientation of adsorbed molecule ν(C-O) CO (gas phase ) 2143 cm-1 Terminal CO 2100 - 1920 cm-1 Bridging (2 f site) 1920 - 1800 cm-1 Bridging (3 f/4 f site) < 1800 cm-1
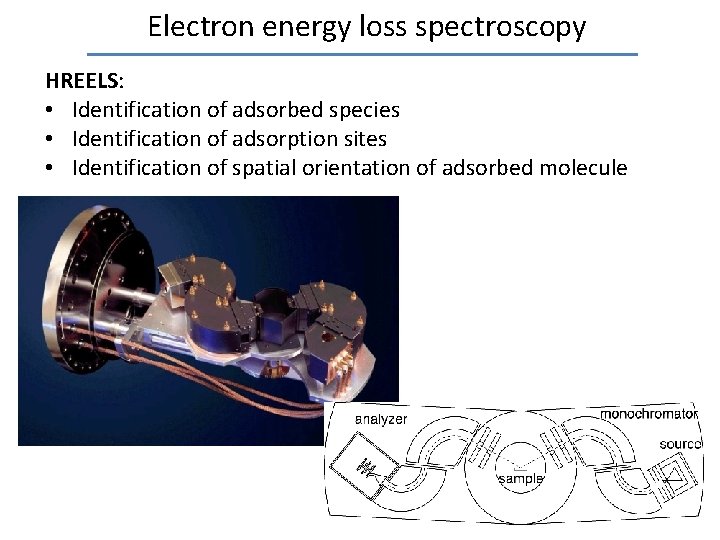
Electron energy loss spectroscopy HREELS: • Identification of adsorbed species • Identification of adsorption sites • Identification of spatial orientation of adsorbed molecule
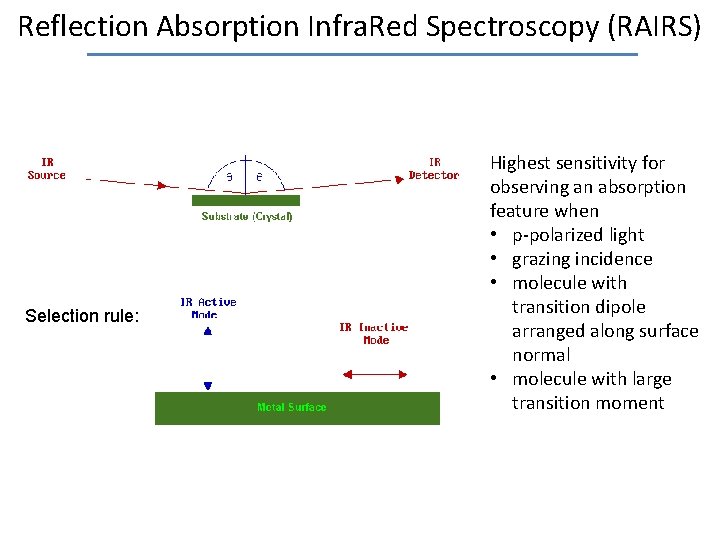
Reflection Absorption Infra. Red Spectroscopy (RAIRS) Selection rule: Highest sensitivity for observing an absorption feature when • p-polarized light • grazing incidence • molecule with transition dipole arranged along surface normal • molecule with large transition moment
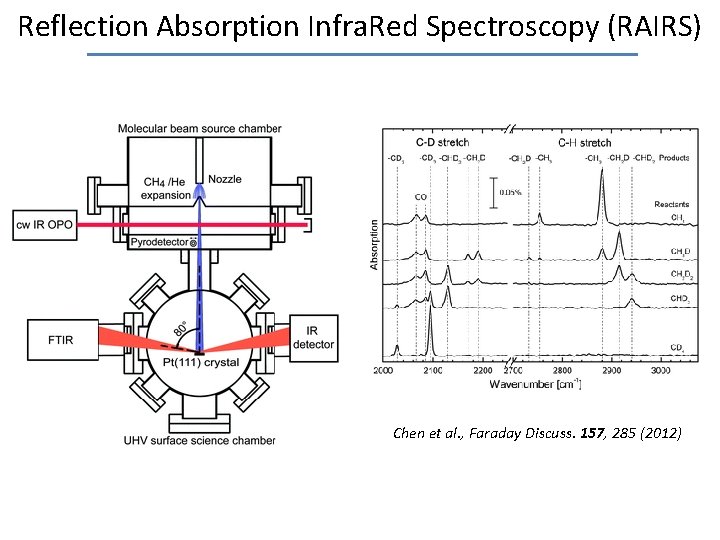
Reflection Absorption Infra. Red Spectroscopy (RAIRS) Chen et al. , Faraday Discuss. 157, 285 (2012)
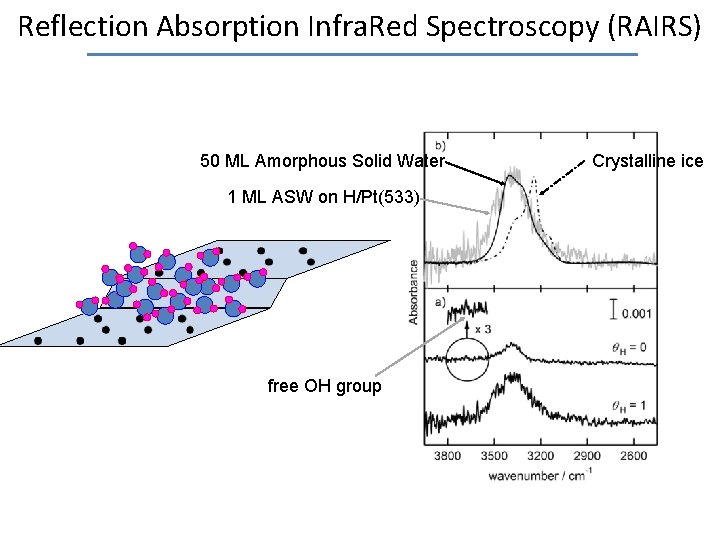
Reflection Absorption Infra. Red Spectroscopy (RAIRS) 50 ML Amorphous Solid Water 1 ML ASW on H/Pt(533) free OH group Crystalline ice
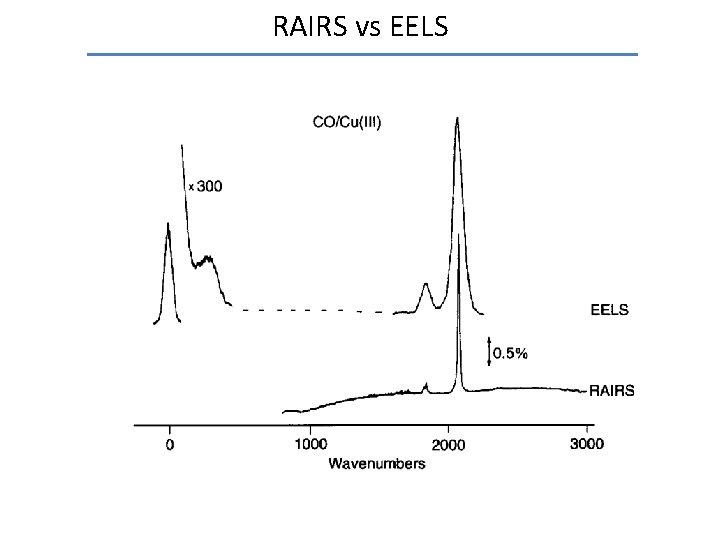
RAIRS vs EELS
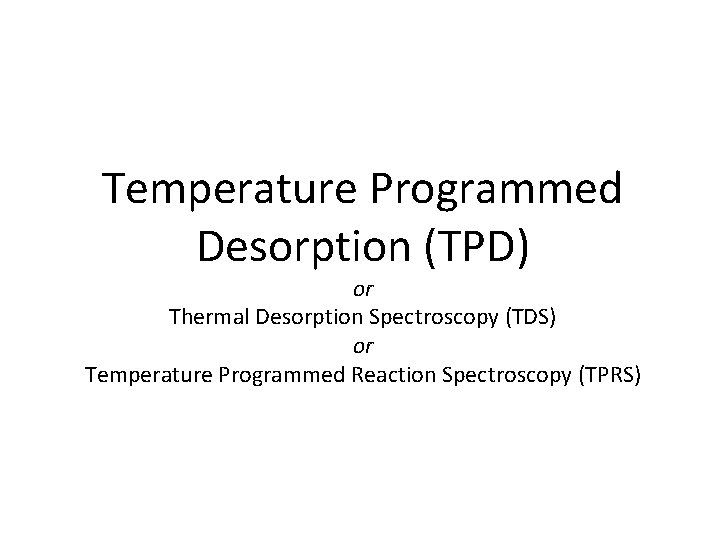
Temperature Programmed Desorption (TPD) or Thermal Desorption Spectroscopy (TDS) or Temperature Programmed Reaction Spectroscopy (TPRS)
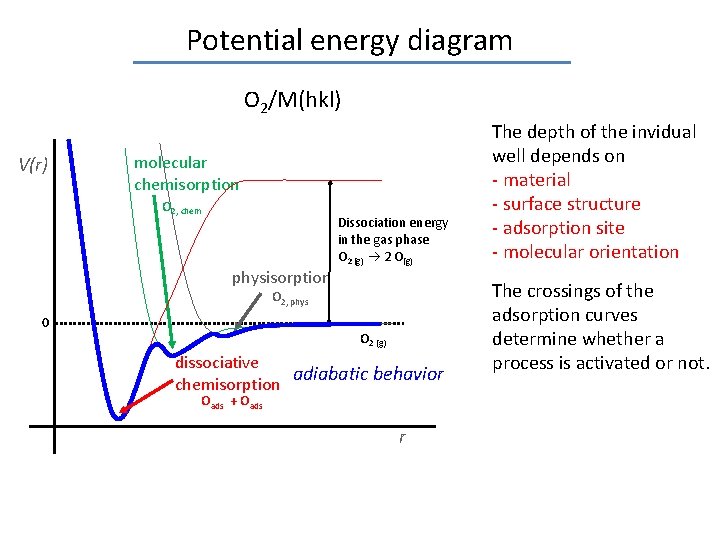
Potential energy diagram O 2/M(hkl) V(r) molecular chemisorption O 2, chem physisorption Dissociation energy in the gas phase O 2 (g) 2 O(g) O 2, phys 0 O 2 (g) dissociative adiabatic behavior chemisorption Oads + Oads r The depth of the invidual well depends on - material - surface structure - adsorption site - molecular orientation The crossings of the adsorption curves determine whether a process is activated or not.
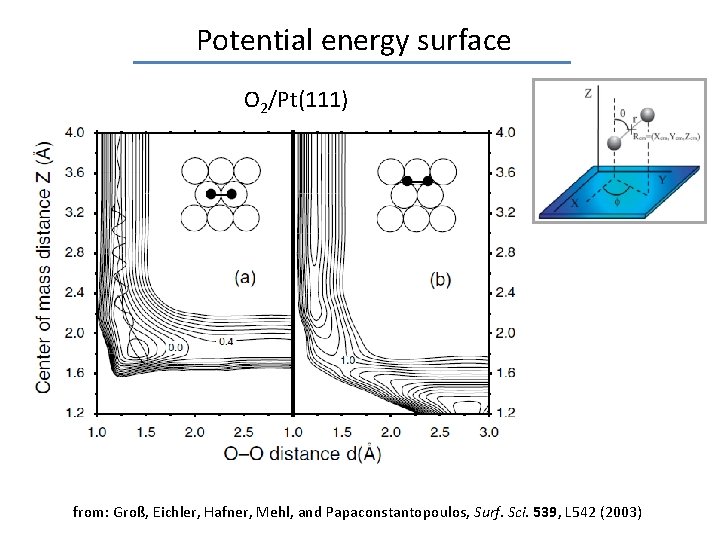
Potential energy surface O 2/Pt(111) from: Groß, Eichler, Hafner, Mehl, and Papaconstantopoulos, Surf. Sci. 539, L 542 (2003)
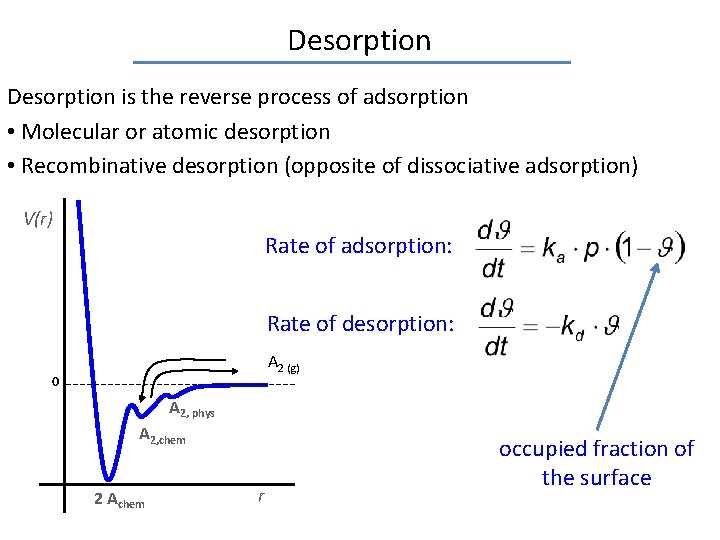
Desorption is the reverse process of adsorption • Molecular or atomic desorption • Recombinative desorption (opposite of dissociative adsorption) V(r) Rate of adsorption: Rate of desorption: A 2 (g) 0 A 2, phys A 2, chem 2 Achem r occupied fraction of the surface
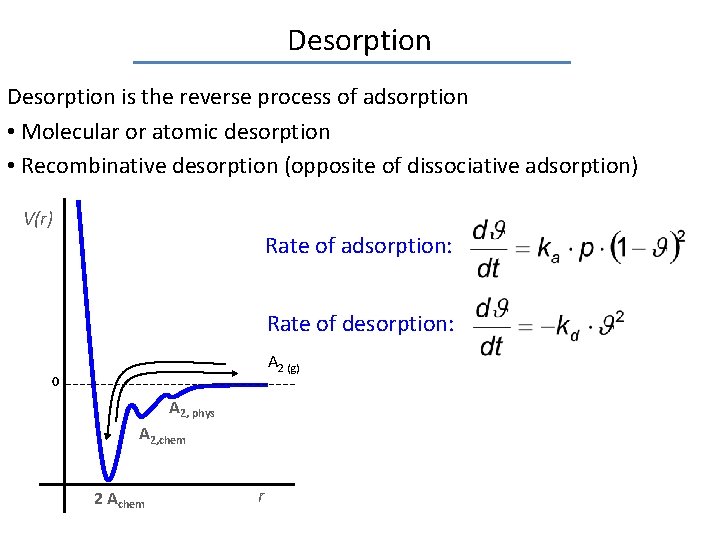
Desorption is the reverse process of adsorption • Molecular or atomic desorption • Recombinative desorption (opposite of dissociative adsorption) V(r) Rate of adsorption: Rate of desorption: A 2 (g) 0 A 2, phys A 2, chem 2 Achem r
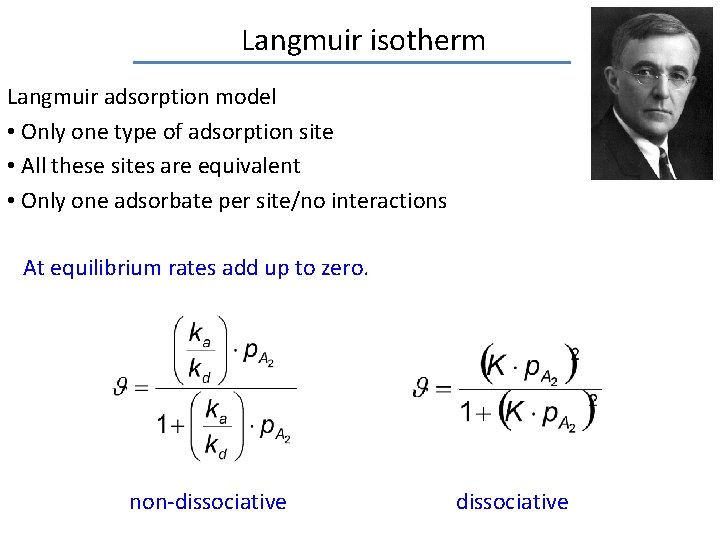
Langmuir isotherm Langmuir adsorption model • Only one type of adsorption site • All these sites are equivalent • Only one adsorbate per site/no interactions At equilibrium rates add up to zero. non-dissociative
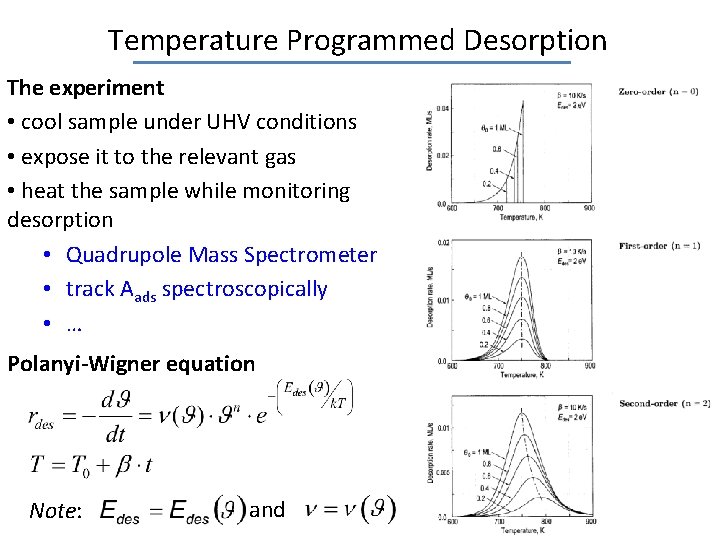
Temperature Programmed Desorption The experiment • cool sample under UHV conditions • expose it to the relevant gas • heat the sample while monitoring desorption • Quadrupole Mass Spectrometer • track Aads spectroscopically • … Polanyi-Wigner equation Note: and
![Example H 2 O interaction with Ptn111x100 Example H 2 O interaction with Pt[n(111)x(100)]](https://slidetodoc.com/presentation_image_h/98256ba861f6f16582625ea403be9ccc/image-35.jpg)
Example H 2 O interaction with Pt[n(111)x(100)]
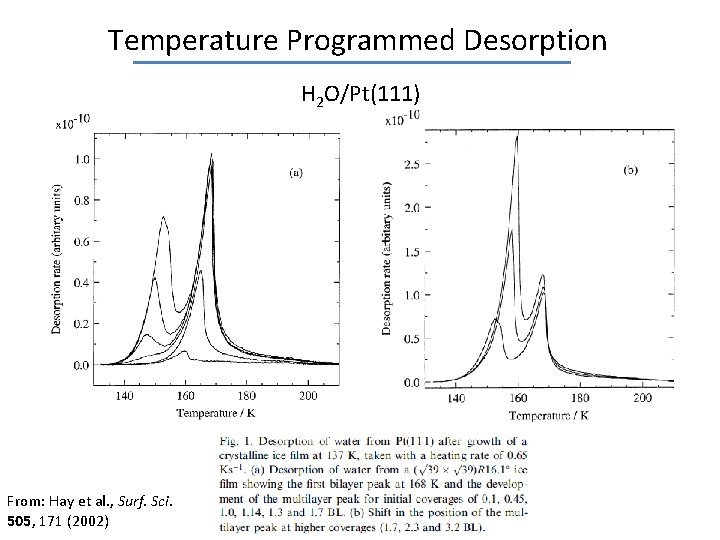
Temperature Programmed Desorption H 2 O/Pt(111) From: Hay et al. , Surf. Sci. 505, 171 (2002)
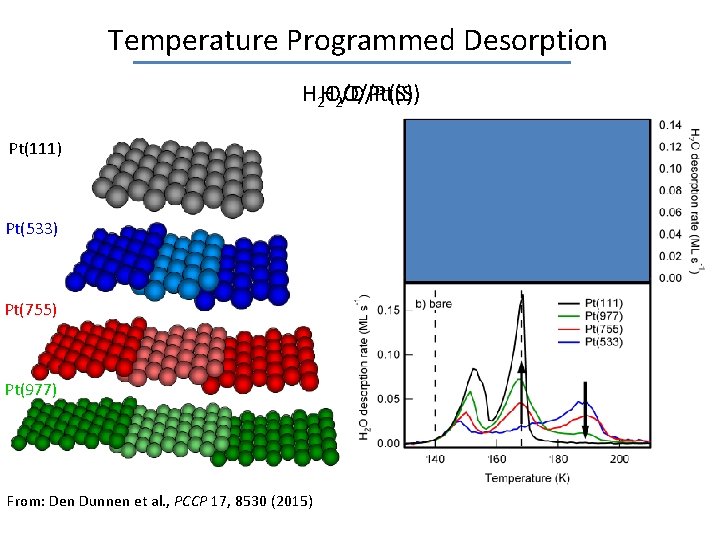
Temperature Programmed Desorption H 2 HO/D/Pt(S) 2 O/Pt(S) Pt(111) Pt(533) Pt(755) Pt(977) From: Den Dunnen et al. , PCCP 17, 8530 (2015)
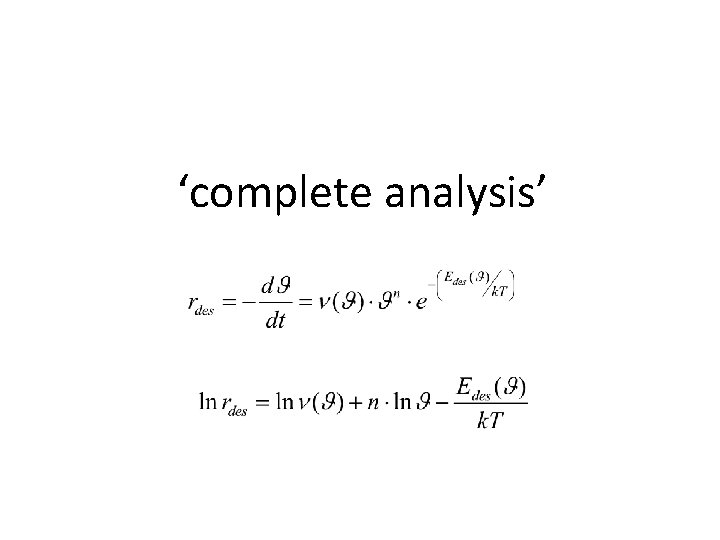
‘complete analysis’
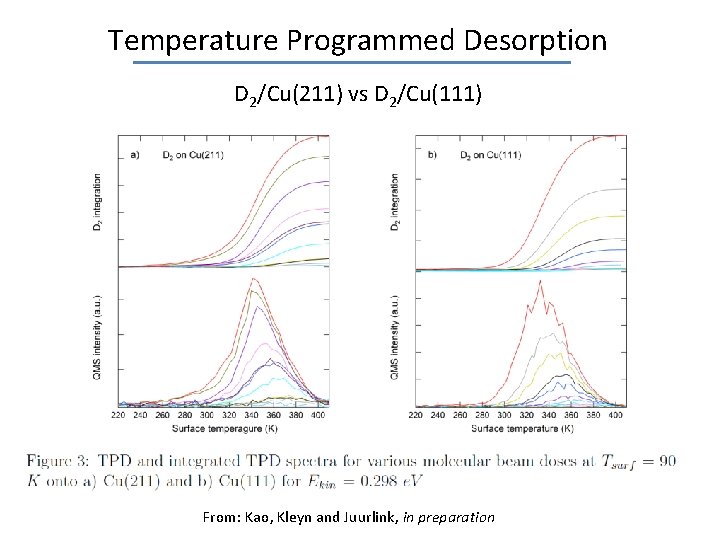
Temperature Programmed Desorption D 2/Cu(211) vs D 2/Cu(111) From: Kao, Kleyn and Juurlink, in preparation
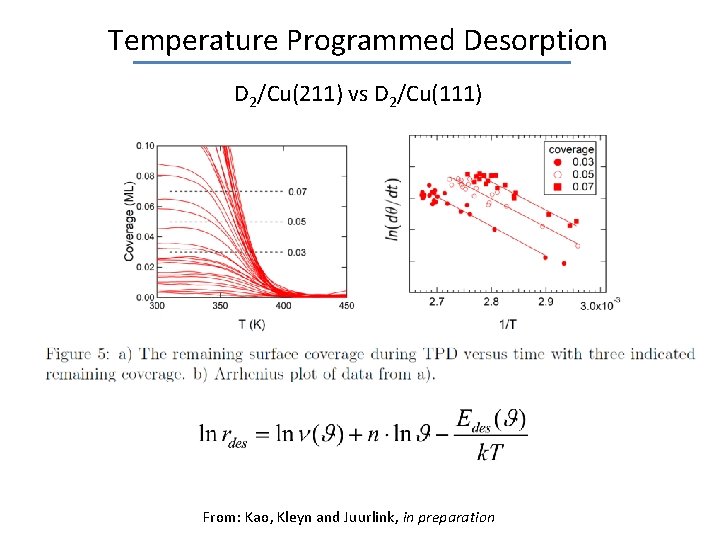
Temperature Programmed Desorption D 2/Cu(211) vs D 2/Cu(111) From: Kao, Kleyn and Juurlink, in preparation
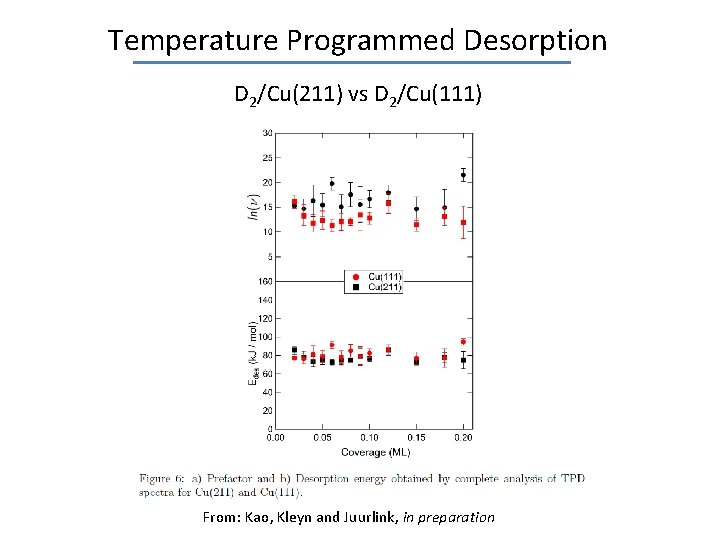
Temperature Programmed Desorption D 2/Cu(211) vs D 2/Cu(111) From: Kao, Kleyn and Juurlink, in preparation
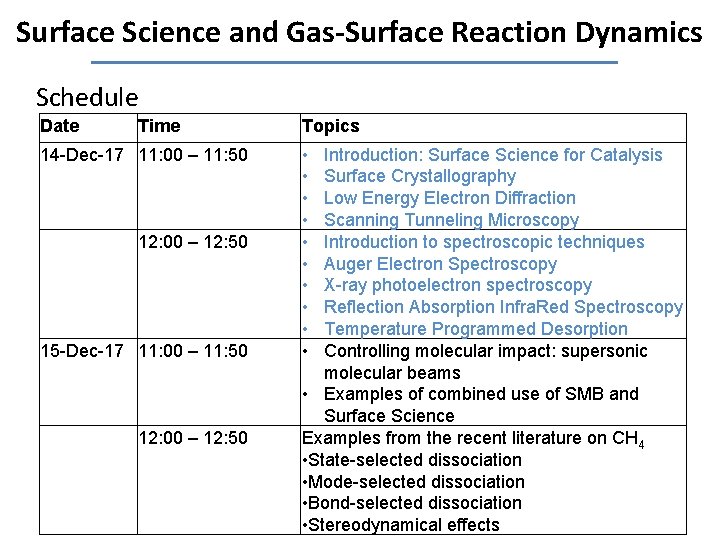
Surface Science and Gas-Surface Reaction Dynamics Schedule Date Time 14 -Dec-17 11: 00 – 11: 50 12: 00 – 12: 50 15 -Dec-17 11: 00 – 11: 50 12: 00 – 12: 50 Topics • • • Introduction: Surface Science for Catalysis Surface Crystallography Low Energy Electron Diffraction Scanning Tunneling Microscopy Introduction to spectroscopic techniques Auger Electron Spectroscopy X-ray photoelectron spectroscopy Reflection Absorption Infra. Red Spectroscopy Temperature Programmed Desorption Controlling molecular impact: supersonic molecular beams • Examples of combined use of SMB and Surface Science Examples from the recent literature on CH 4 • State-selected dissociation • Mode-selected dissociation • Bond-selected dissociation • Stereodynamical effects
Spectroscopic notation
Spectroscopic data in chemistry
Free ion configuration
Limitations of orgel diagram
Two types of physical
Benefits of skill related fitness
My favourite subject is english
Curved surface area and total surface area of cone
What does lateral surface area mean
Fonction technique scooter
High surface tension vs low surface tension
Kontinuitetshantering i praktiken
Typiska novell drag
Nationell inriktning för artificiell intelligens
Returpilarna
Shingelfrisyren
En lathund för arbete med kontinuitetshantering
Personalliggare bygg undantag
Personlig tidbok
Anatomi organ reproduksi
Densitet vatten
Datorkunskap för nybörjare
Stig kerman
Debattartikel mall
För och nackdelar med firo
Nyckelkompetenser för livslångt lärande
Påbyggnader för flakfordon
Vätsketryck formel
Offentlig förvaltning
Kyssande vind
Presentera för publik crossboss
Jiddisch
Plats för toran ark
Treserva lathund
Epiteltyper
Claes martinsson
Cks
Byggprocessen steg för steg
Mat för idrottare
Verktyg för automatisering av utbetalningar
Rutin för avvikelsehantering
Smärtskolan kunskap för livet
Ministerstyre för och nackdelar