Quantum Physics of LightMatter Interactions So Se 20
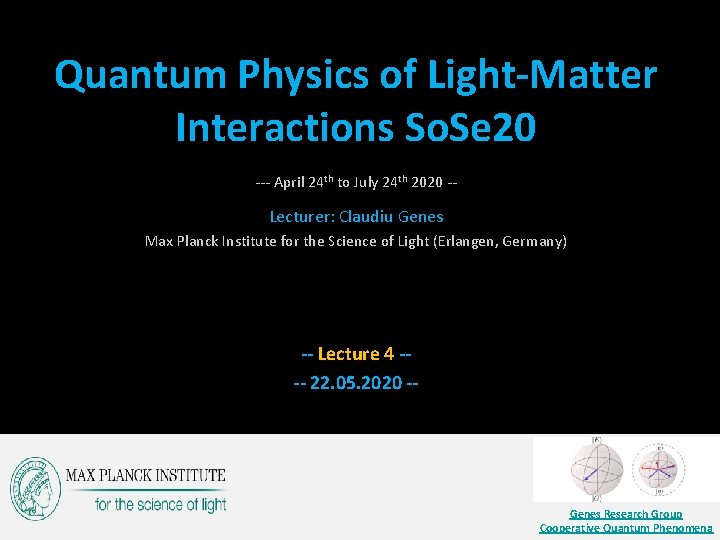
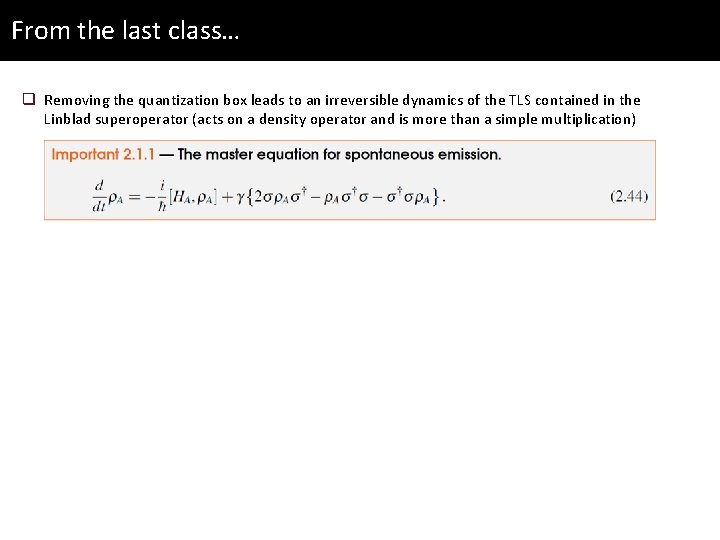
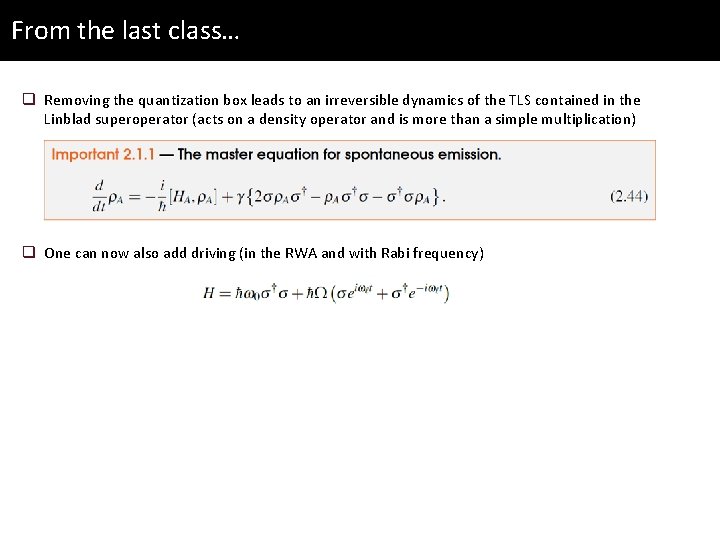
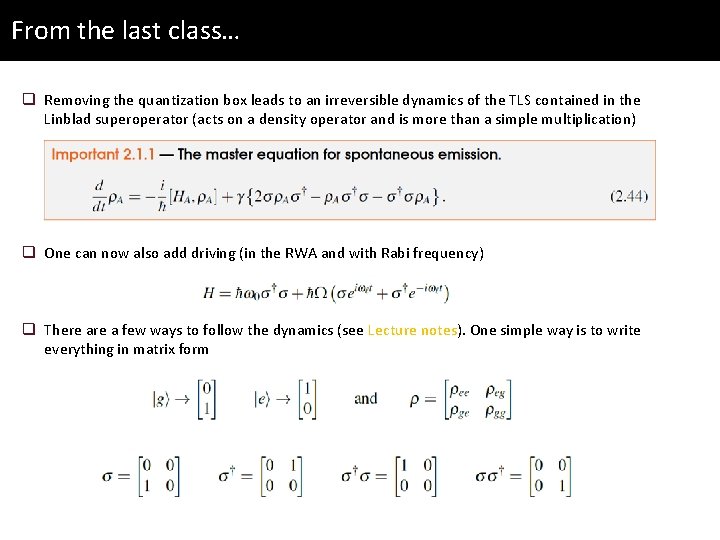
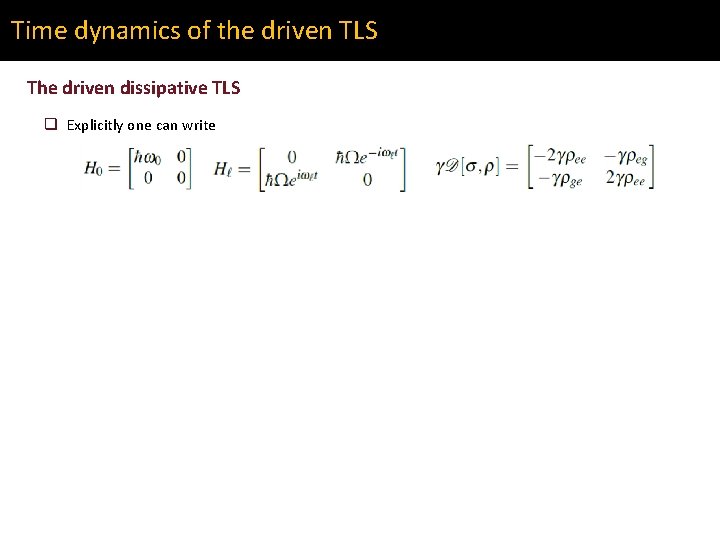
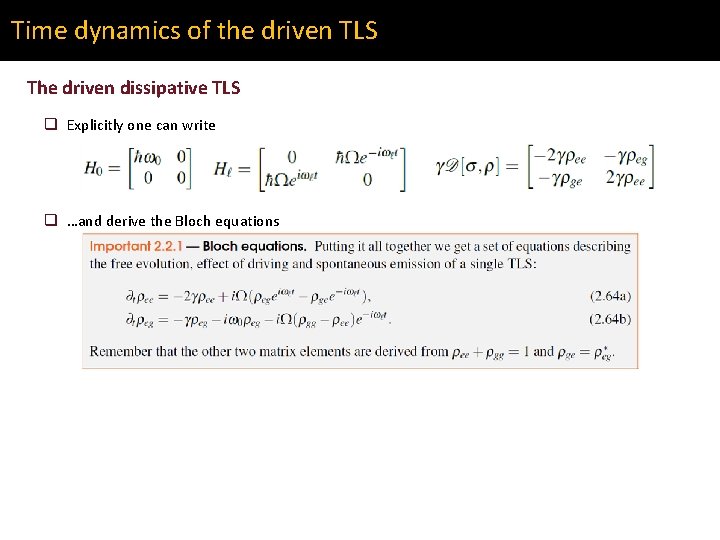
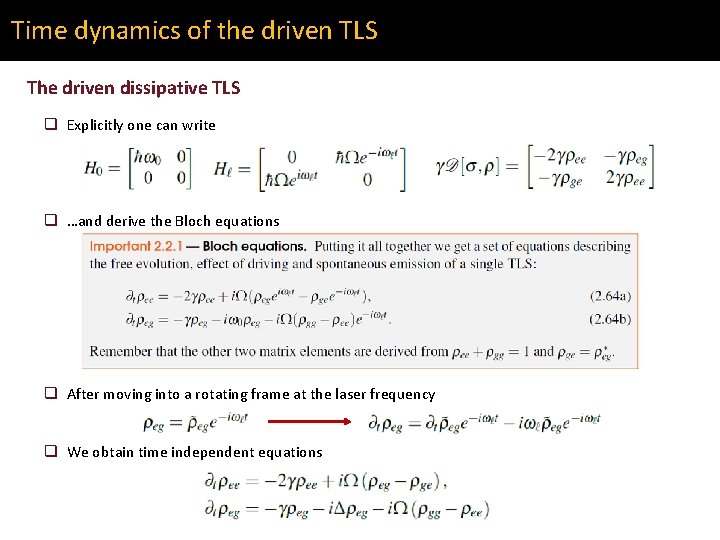
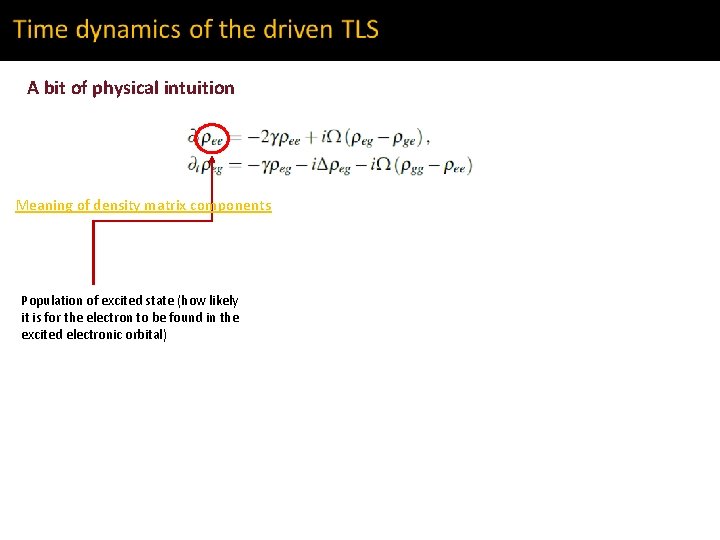
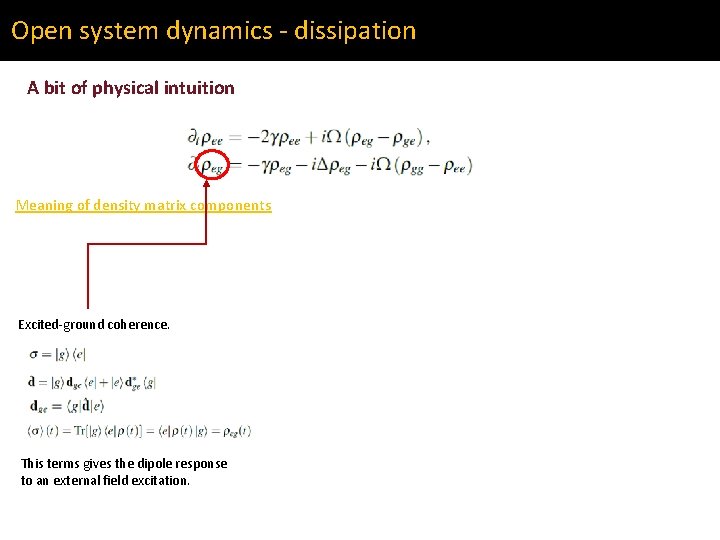
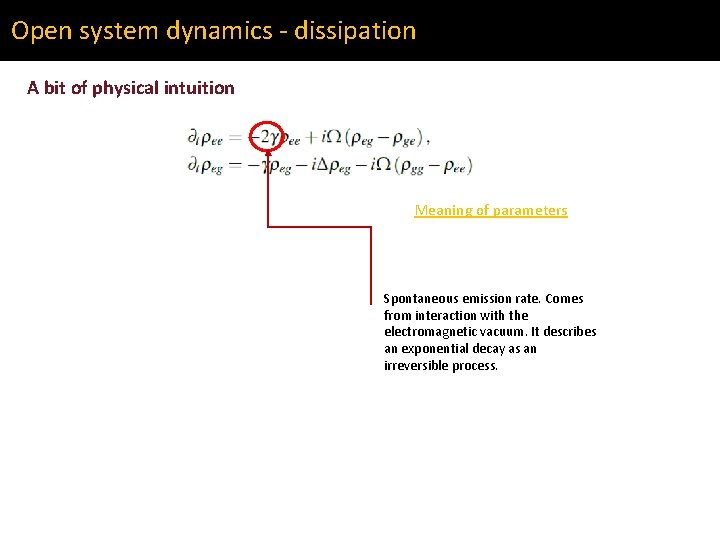
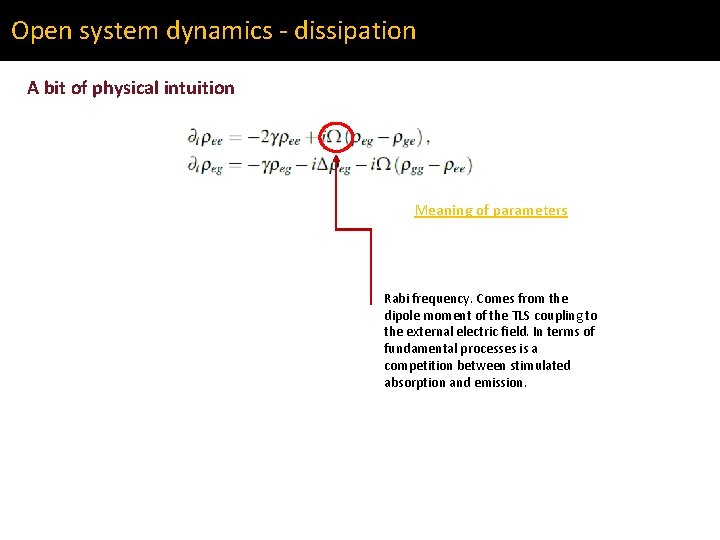
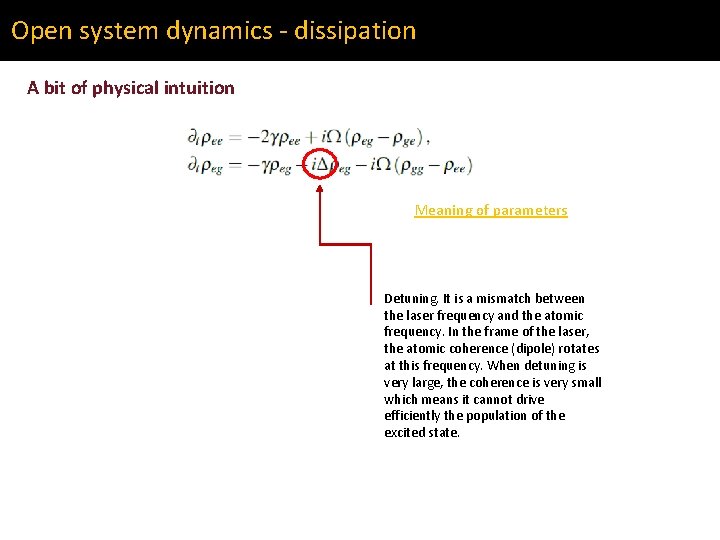
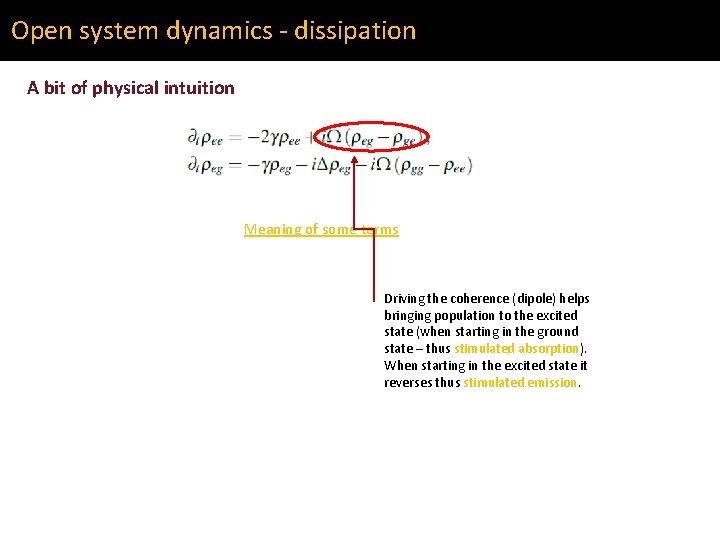
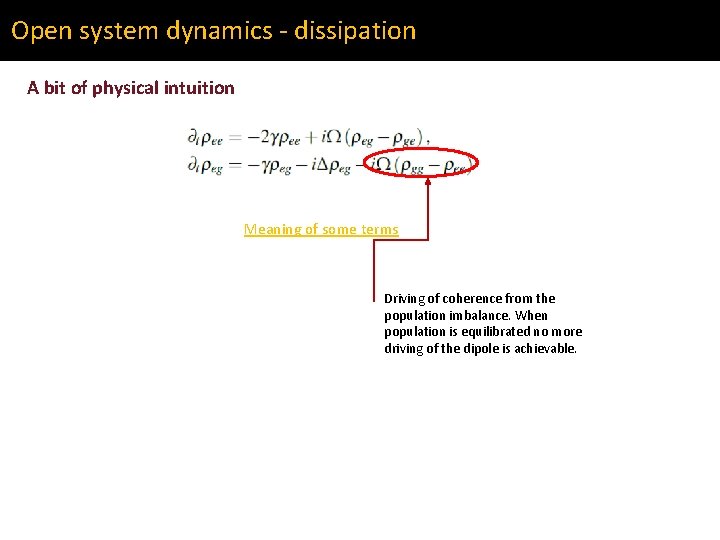
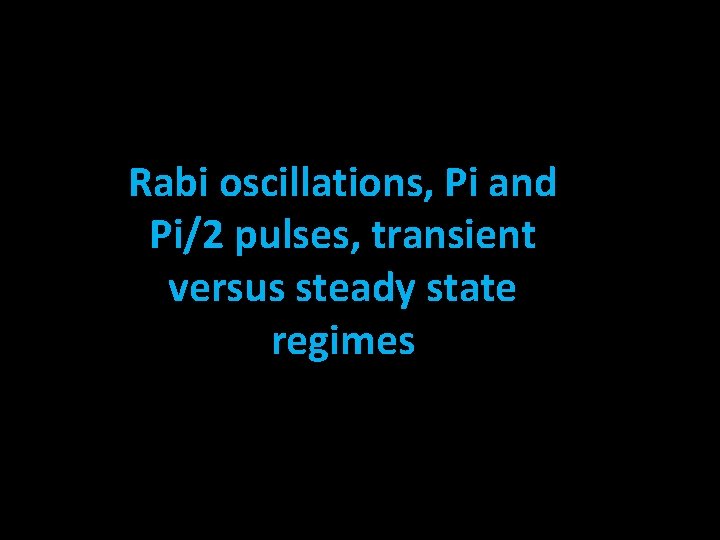
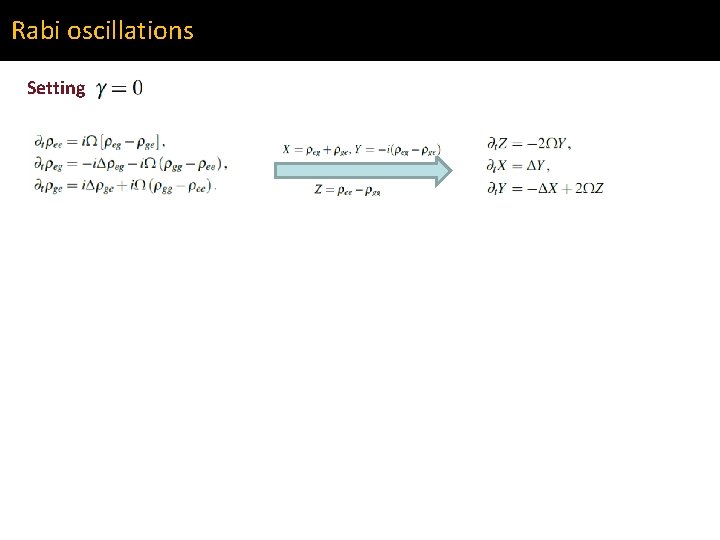
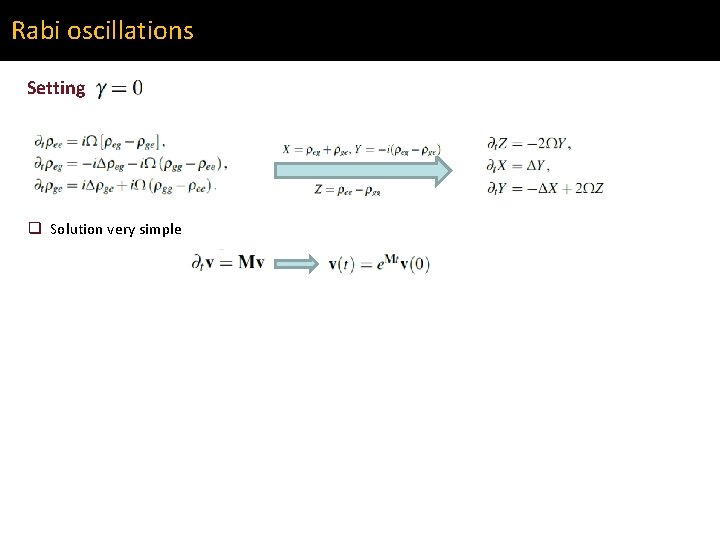
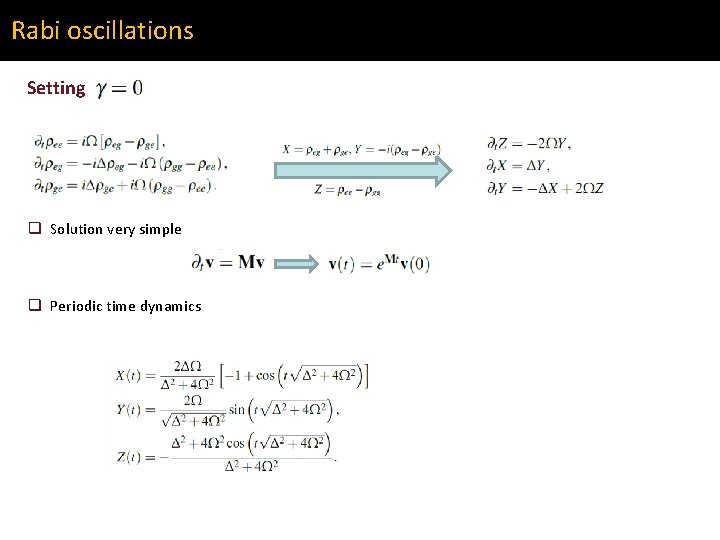
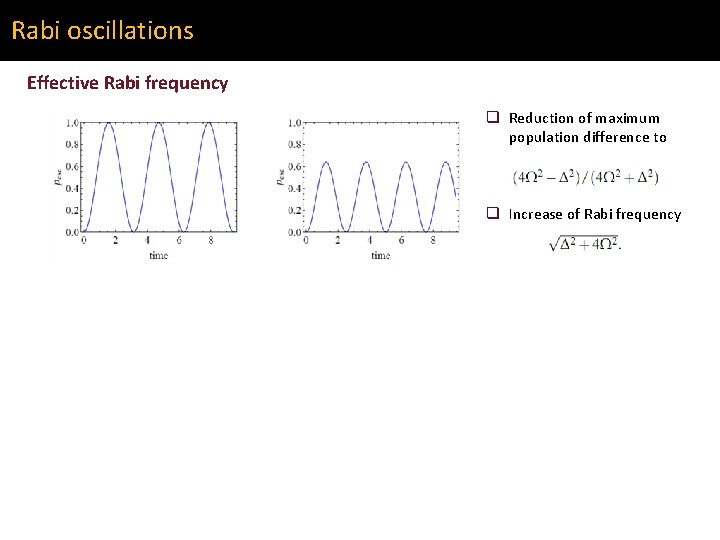
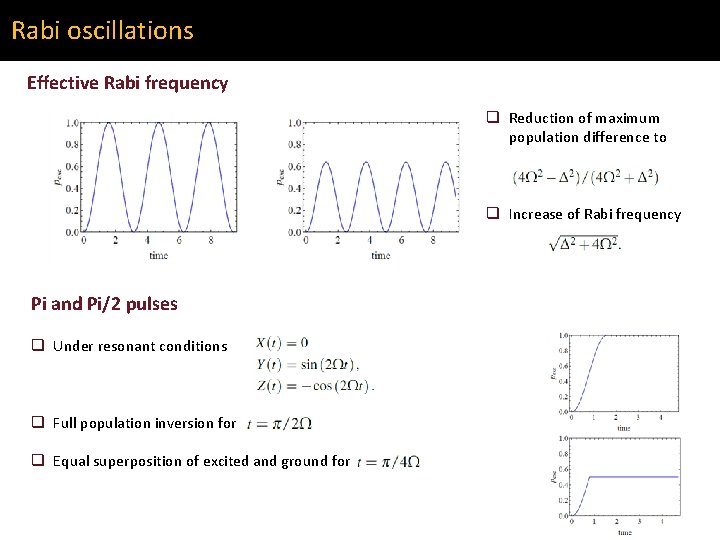
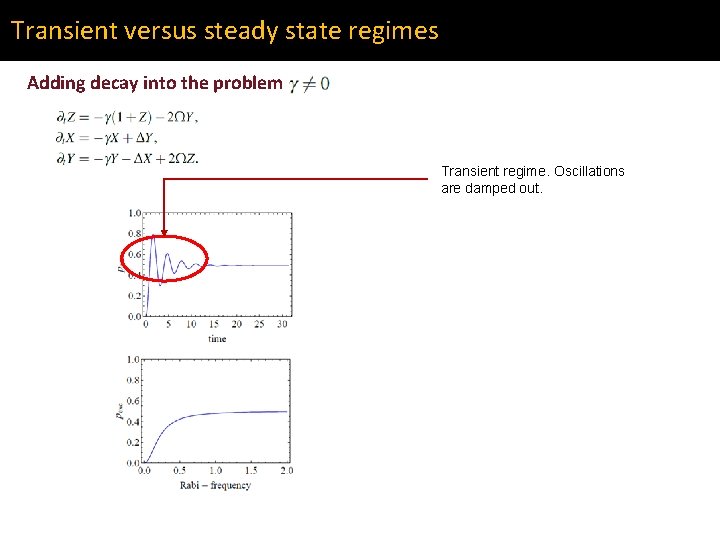
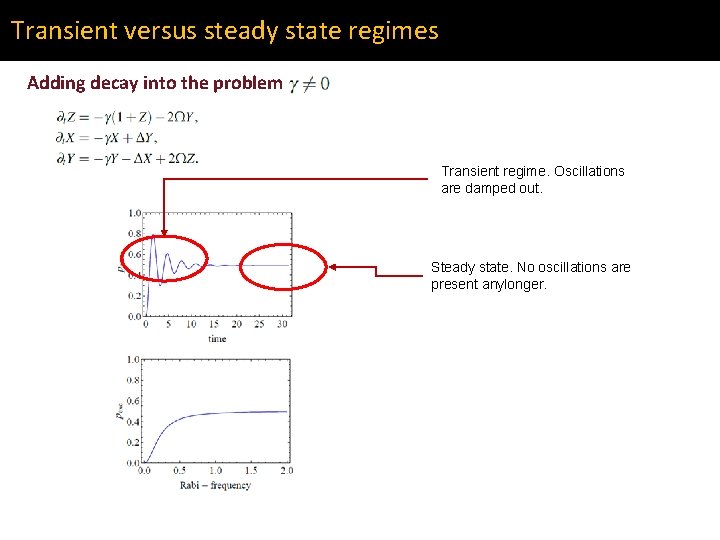
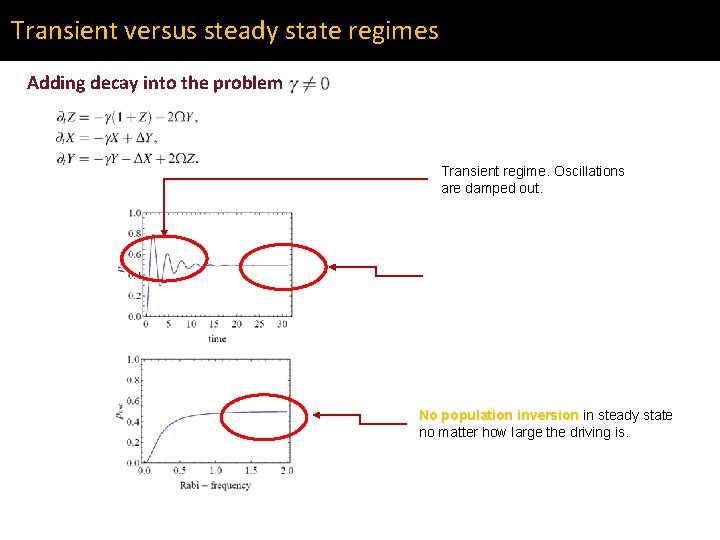
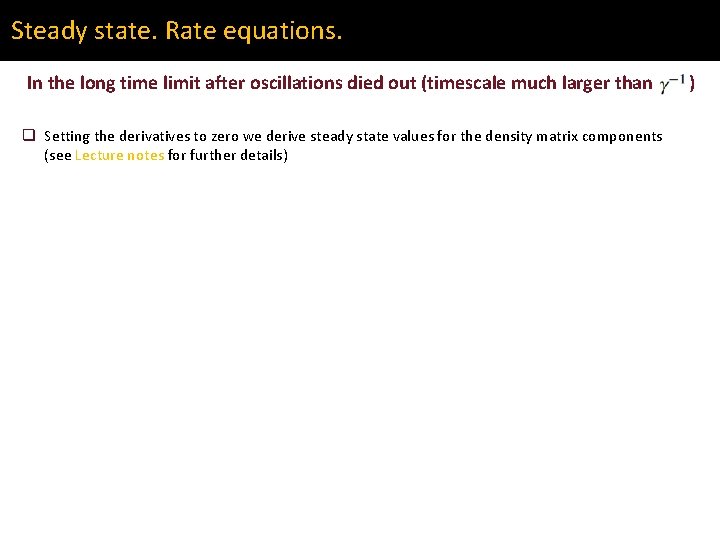
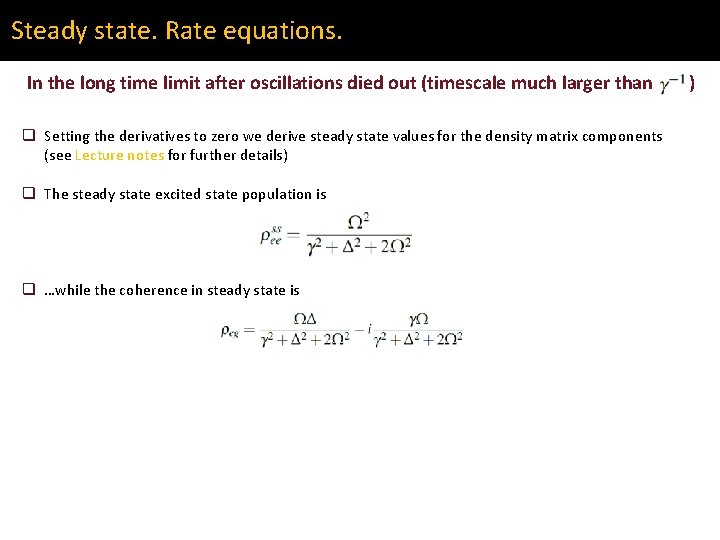
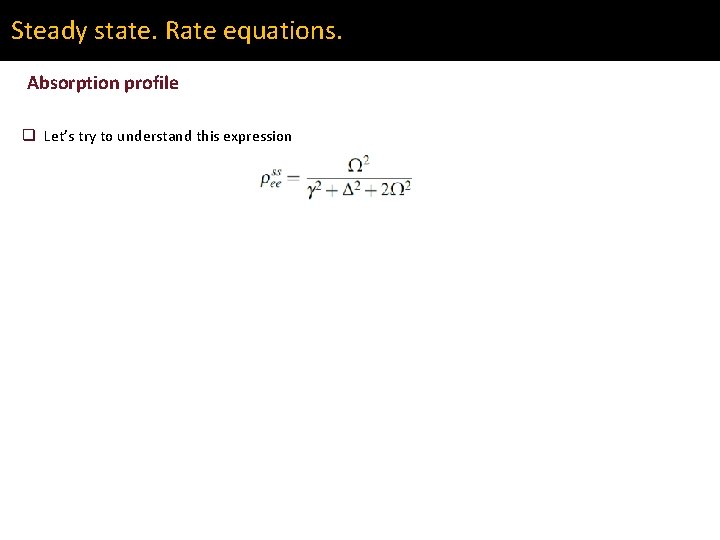
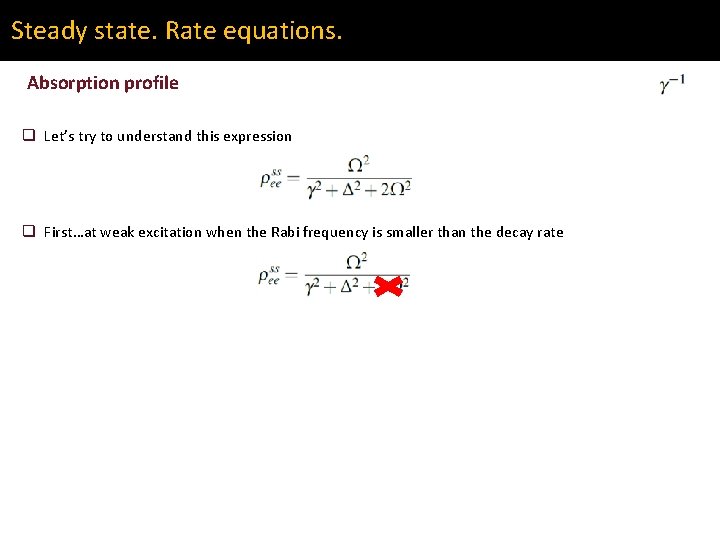
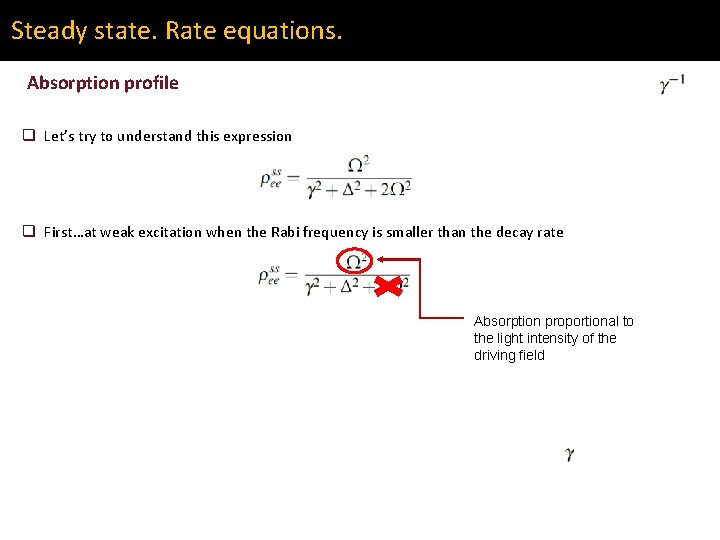
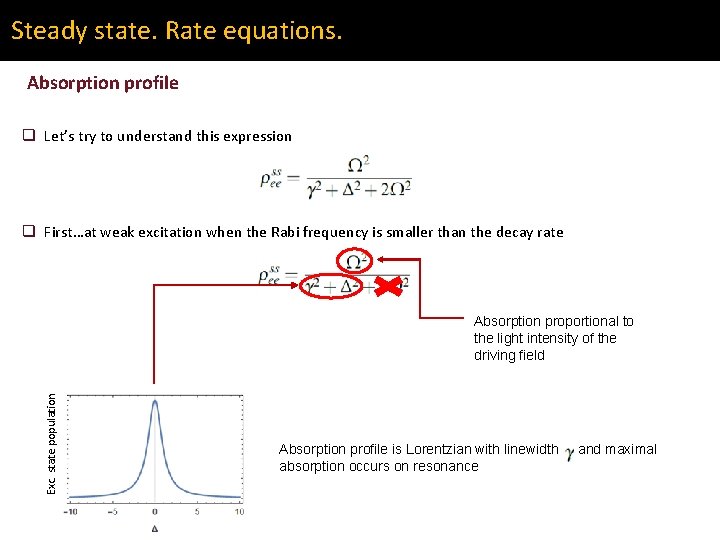
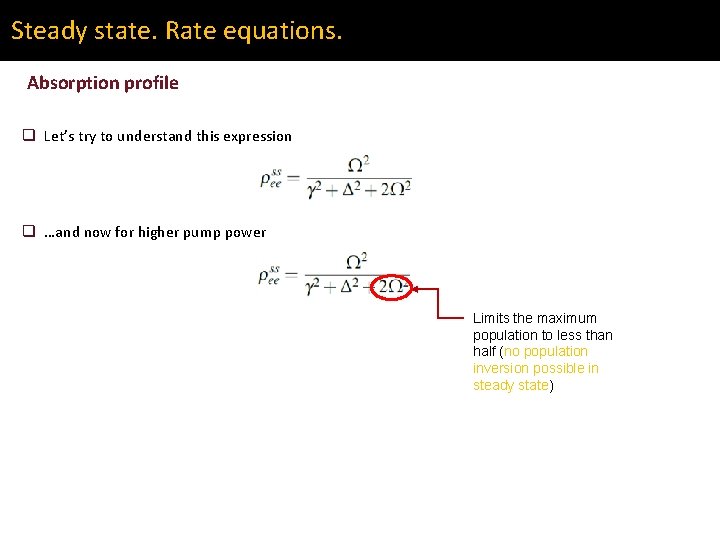
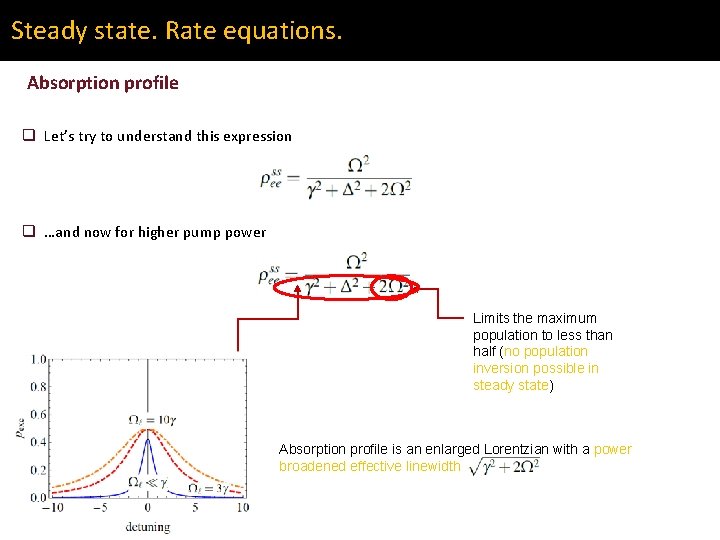
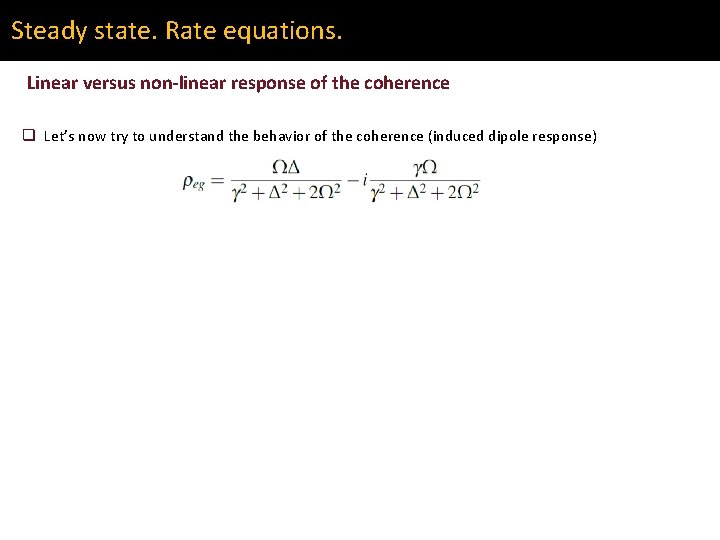
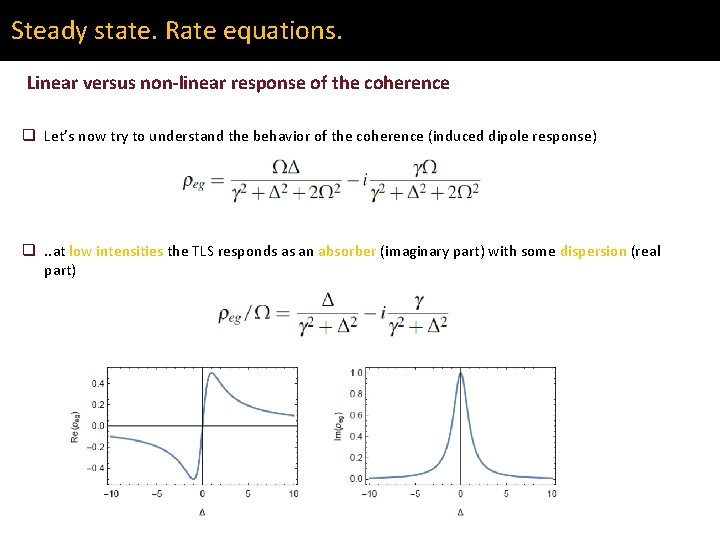
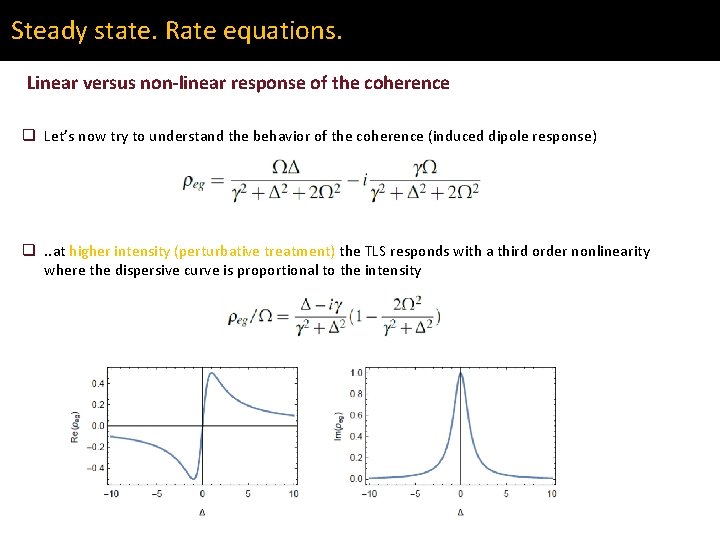
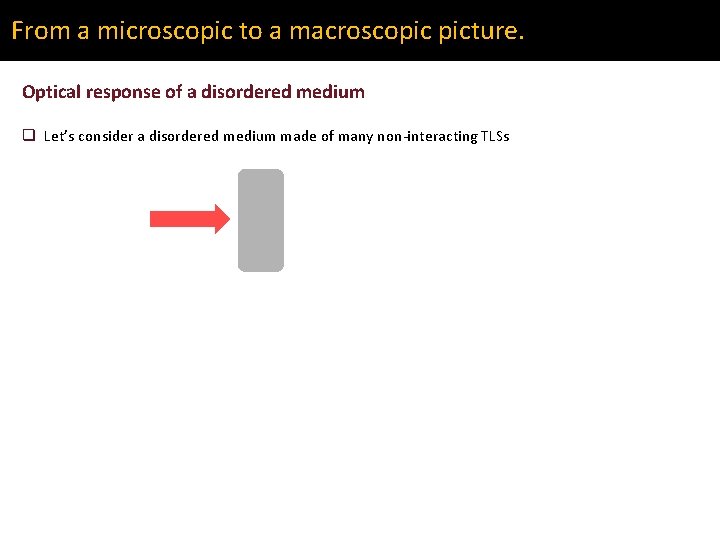
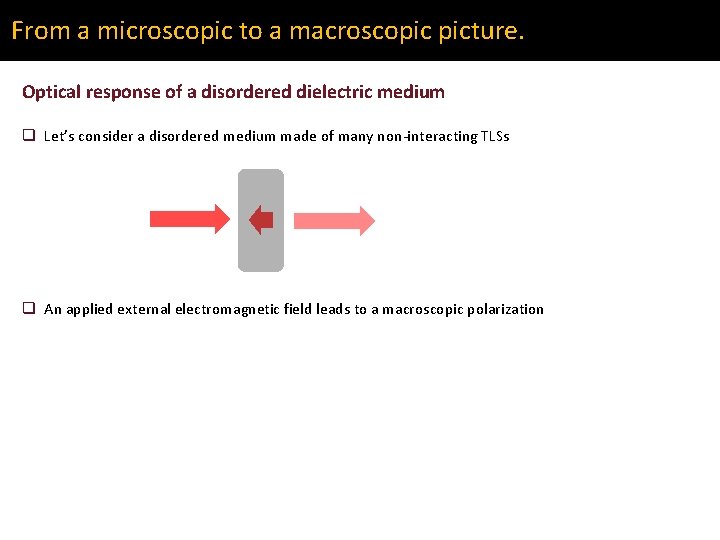
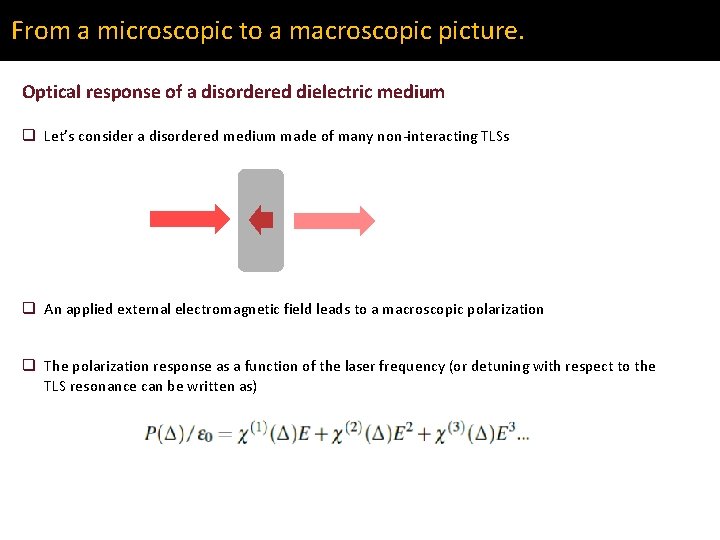
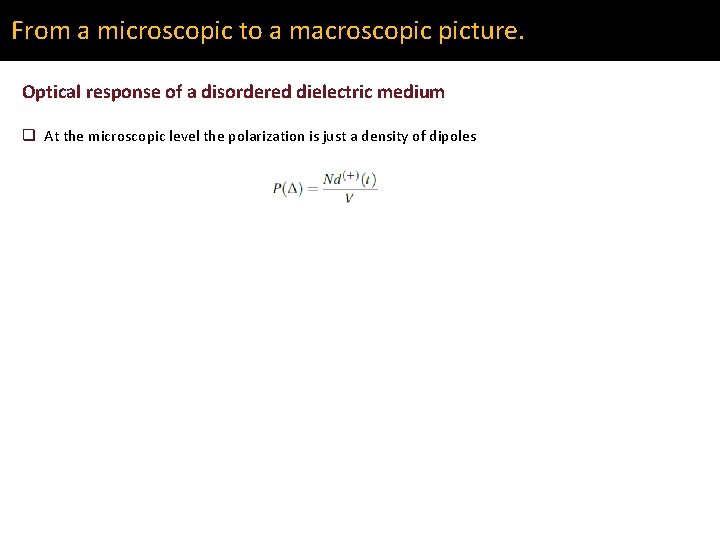
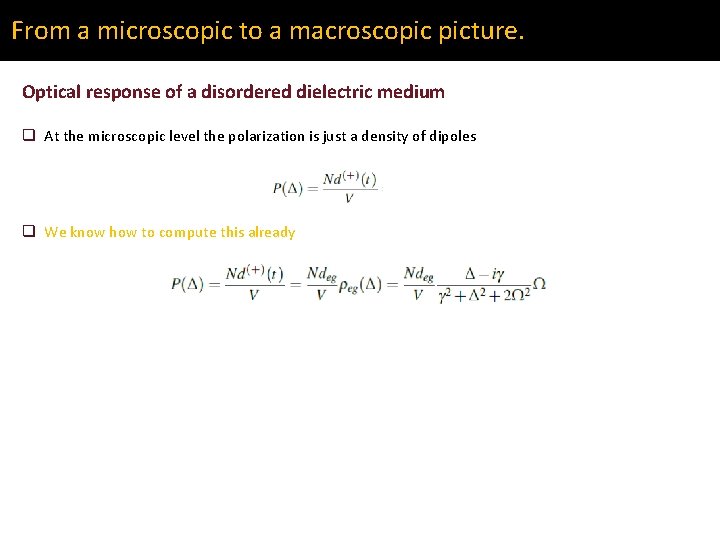
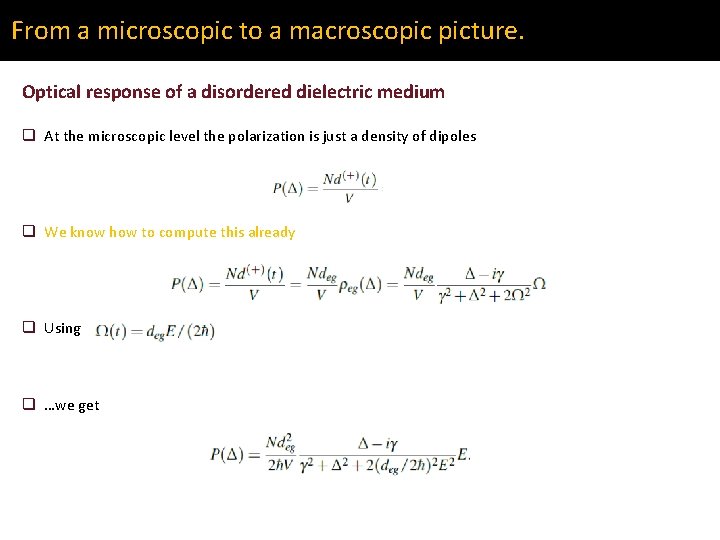
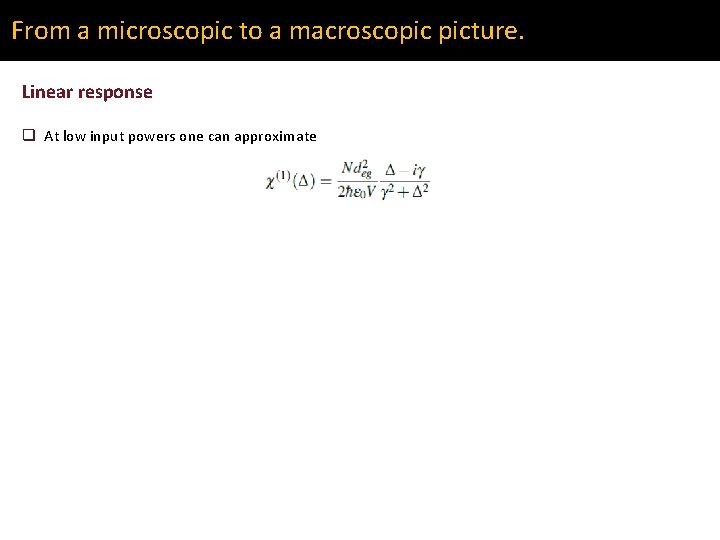
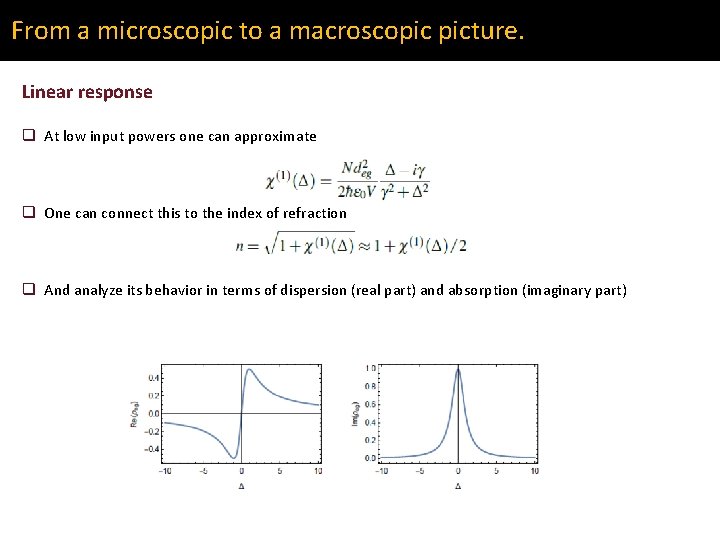
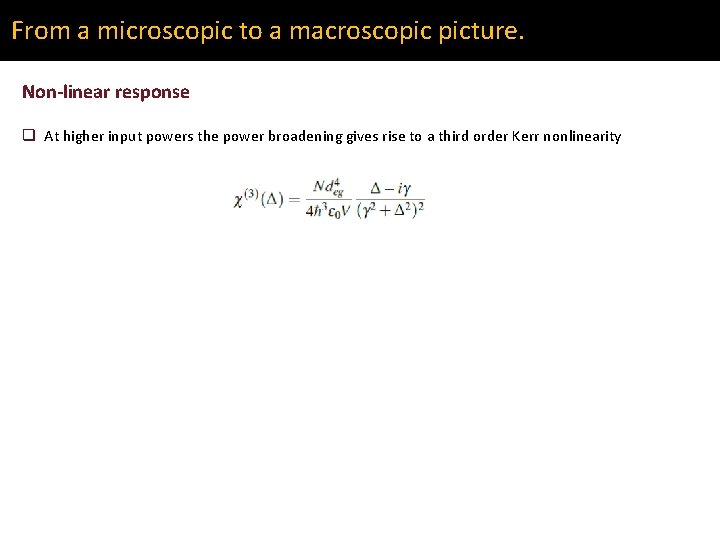
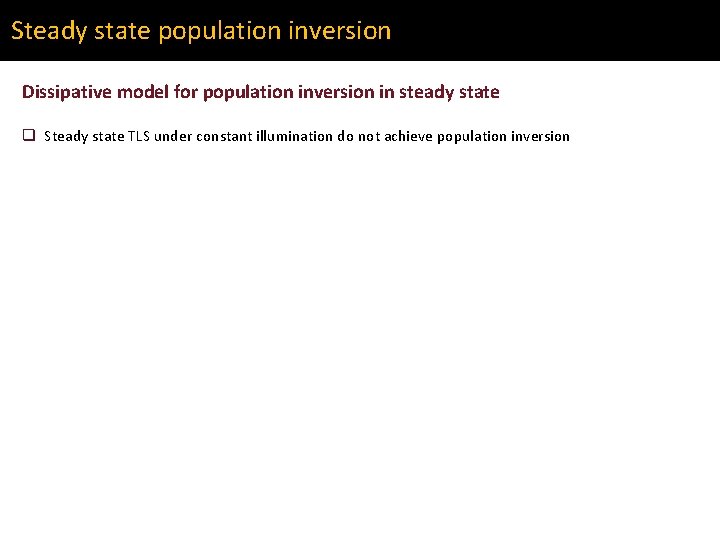
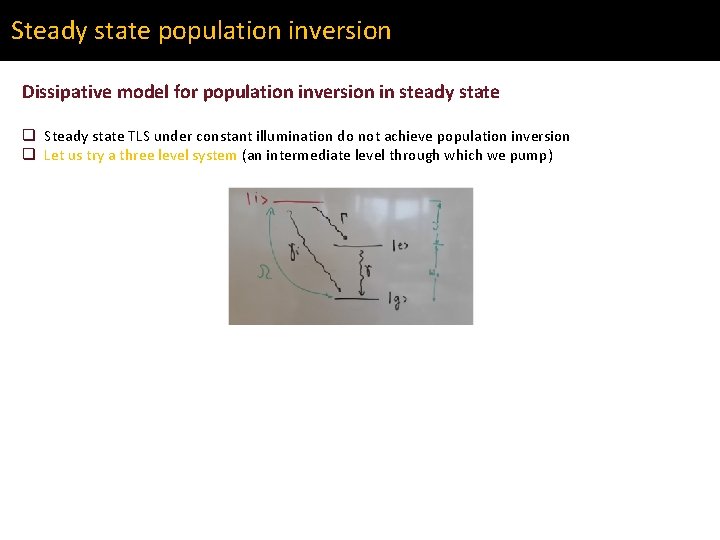
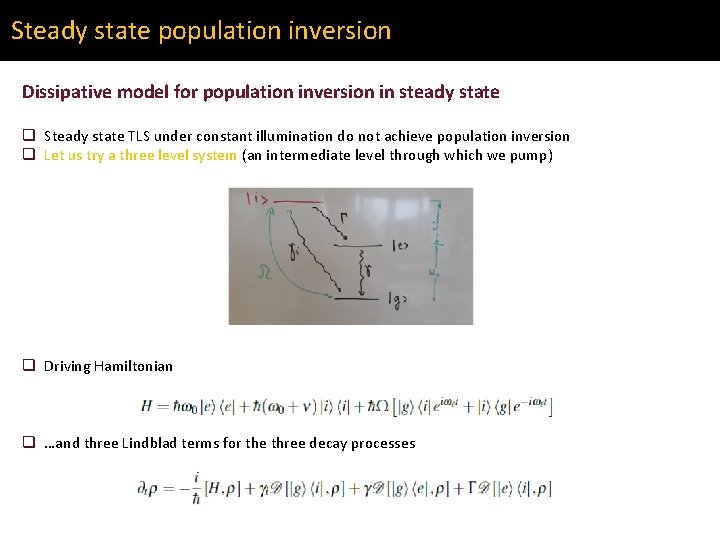
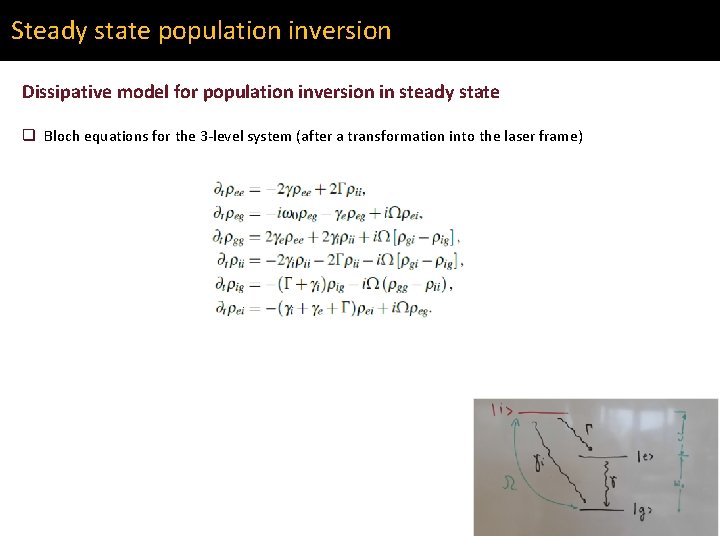
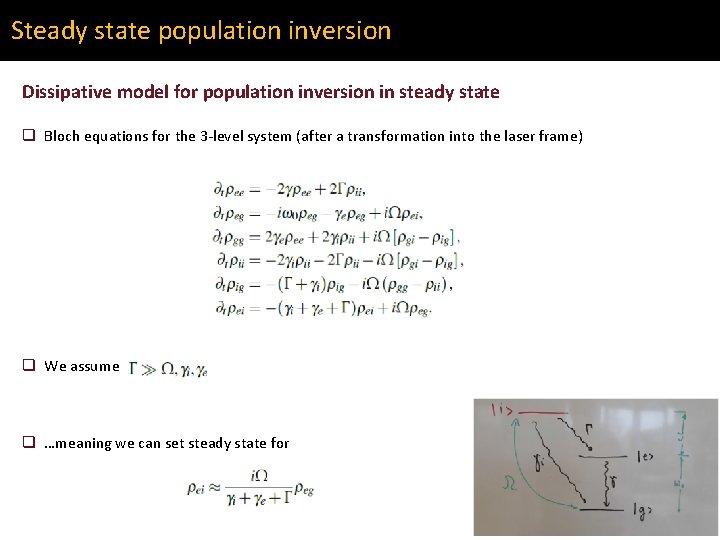
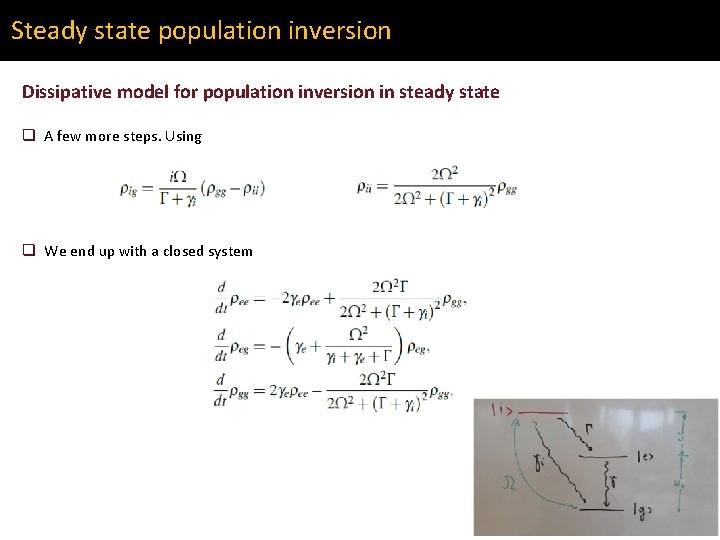
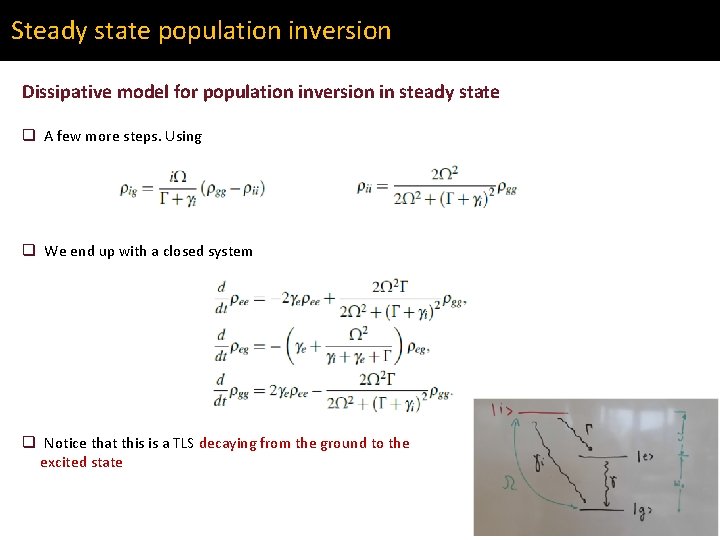
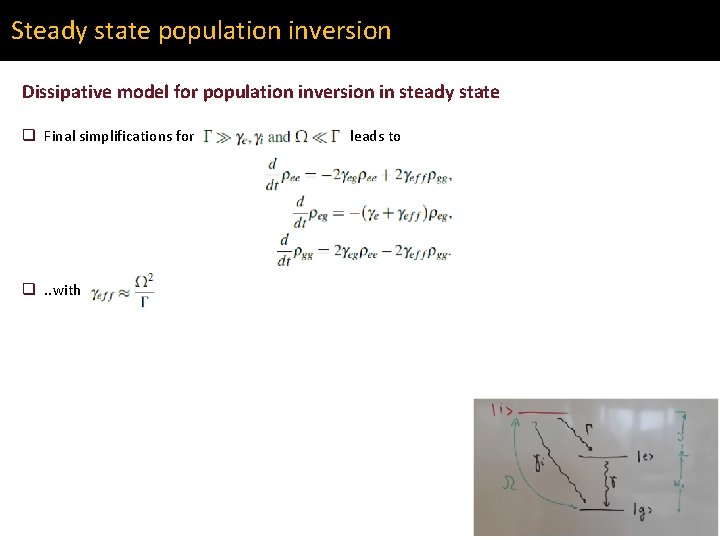
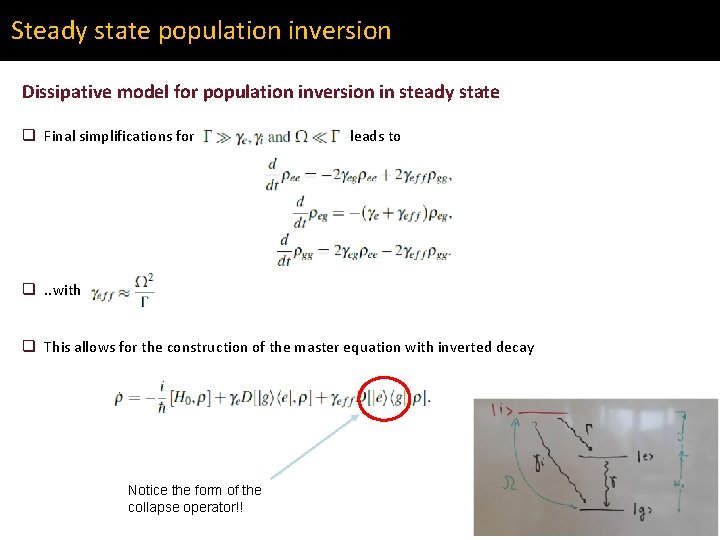
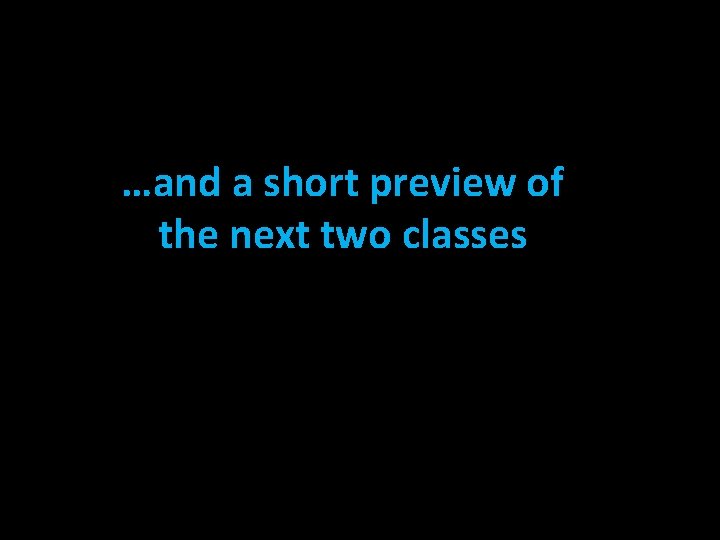
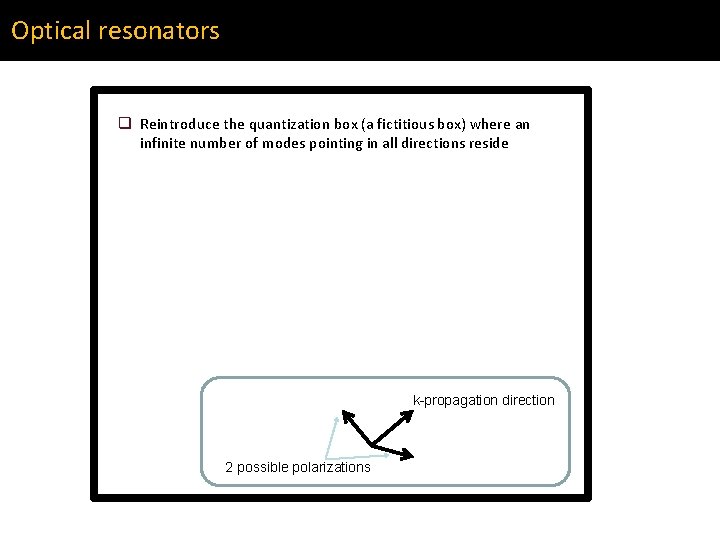
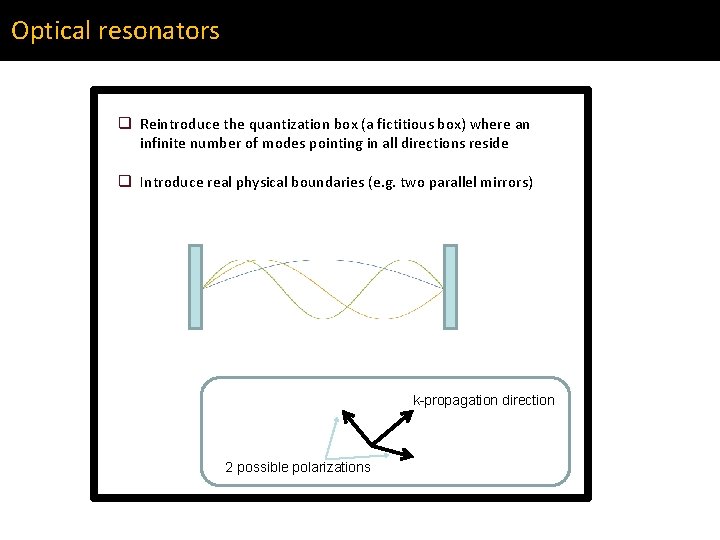
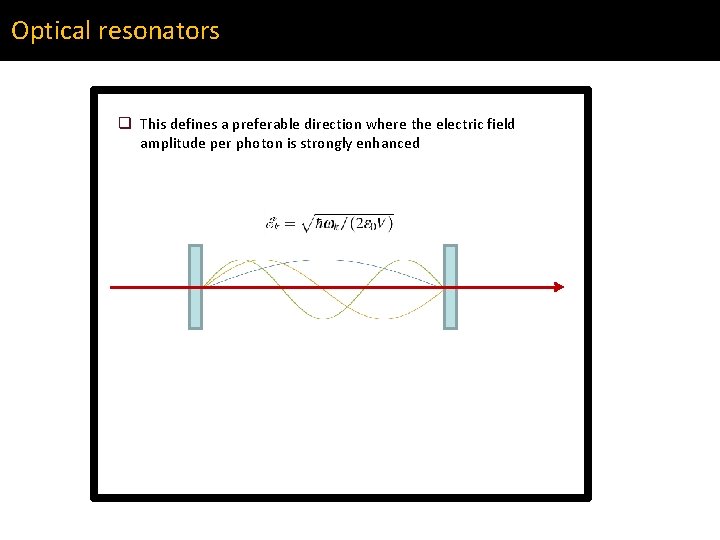
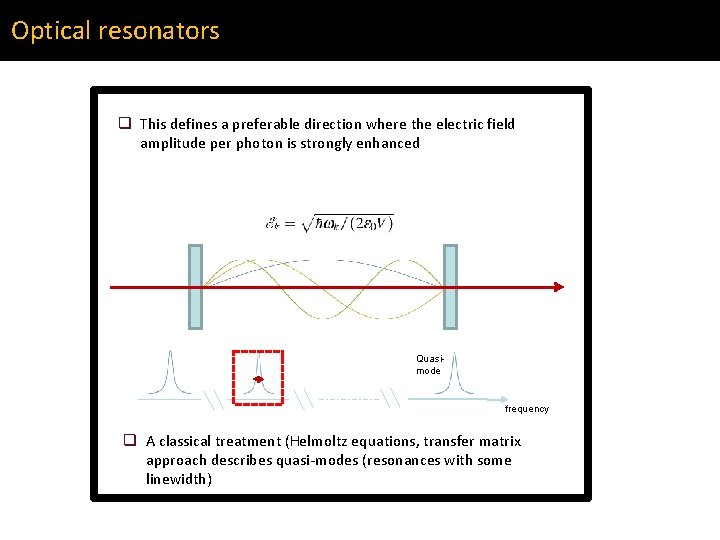
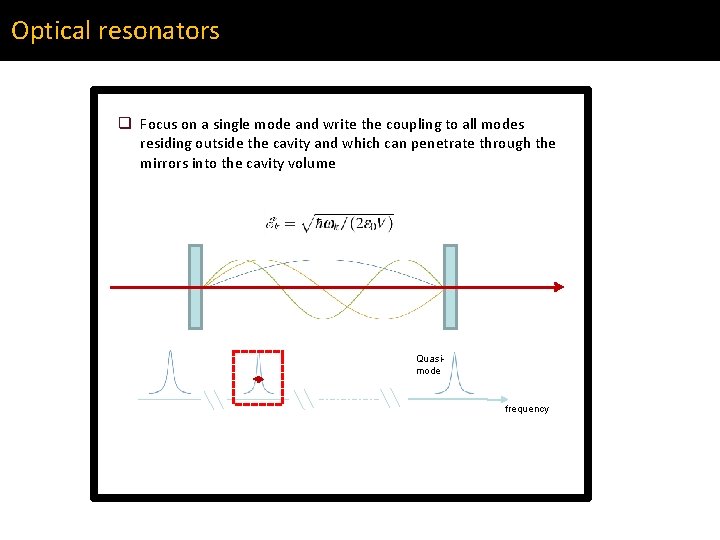
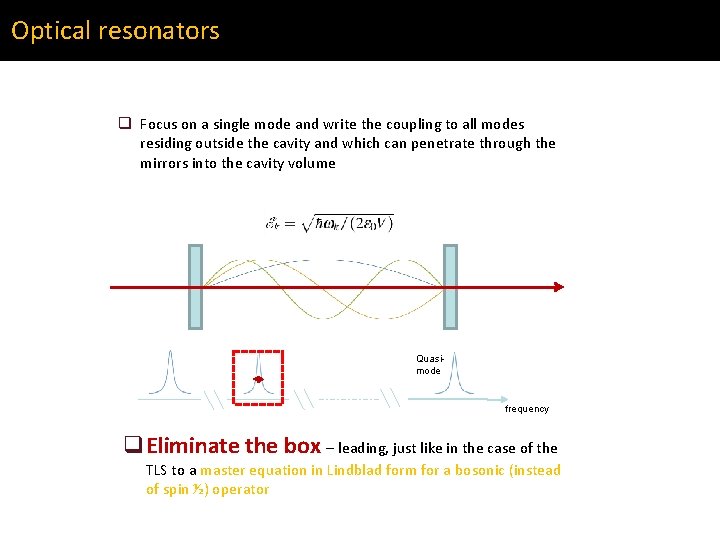
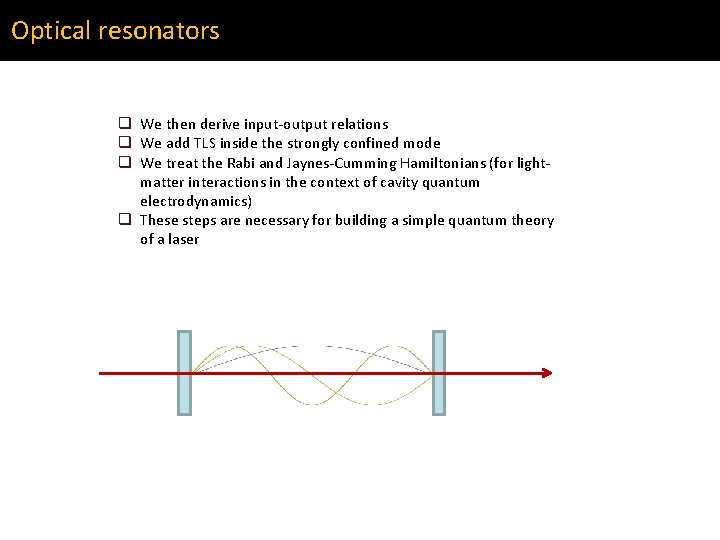
- Slides: 60
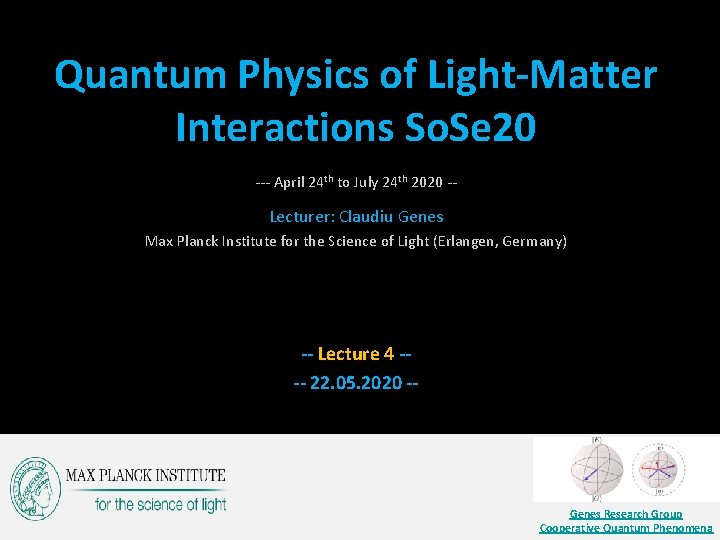
Quantum Physics of Light-Matter Interactions So. Se 20 --- April 24 th to July 24 th 2020 -- Lecturer: Claudiu Genes Max Planck Institute for the Science of Light (Erlangen, Germany) -- Lecture 4 --- 22. 05. 2020 -- Genes Research Group Cooperative Quantum Phenomena
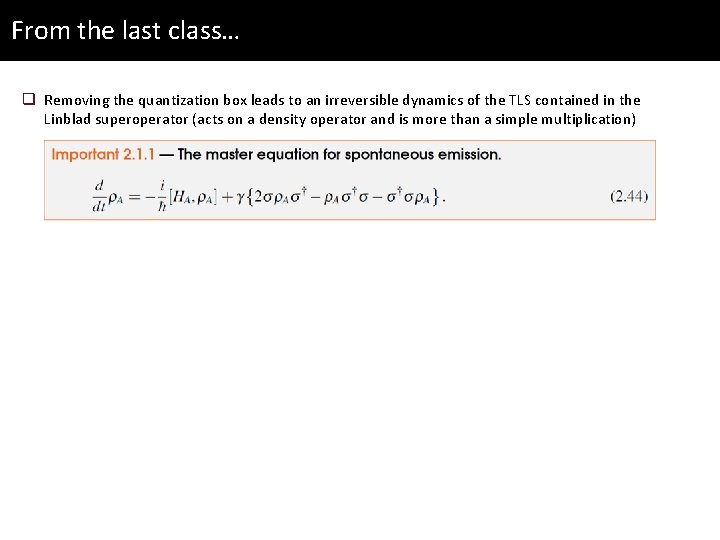
From the last class… q Removing the quantization box leads to an irreversible dynamics of the TLS contained in the Linblad superoperator (acts on a density operator and is more than a simple multiplication)
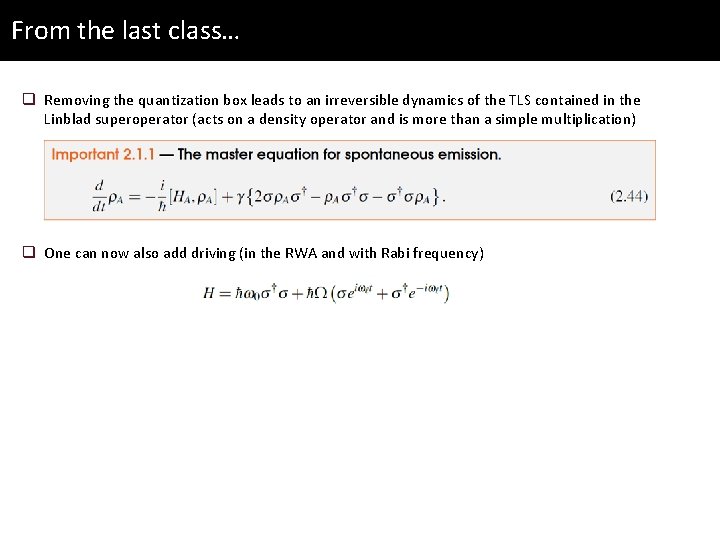
From the last class… q Removing the quantization box leads to an irreversible dynamics of the TLS contained in the Linblad superoperator (acts on a density operator and is more than a simple multiplication) q One can now also add driving (in the RWA and with Rabi frequency)
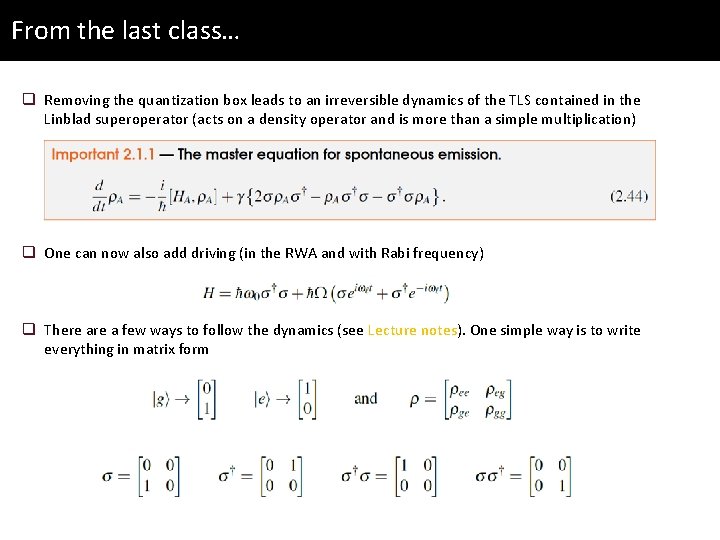
From the last class… q Removing the quantization box leads to an irreversible dynamics of the TLS contained in the Linblad superoperator (acts on a density operator and is more than a simple multiplication) q One can now also add driving (in the RWA and with Rabi frequency) q There a few ways to follow the dynamics (see Lecture notes). One simple way is to write everything in matrix form
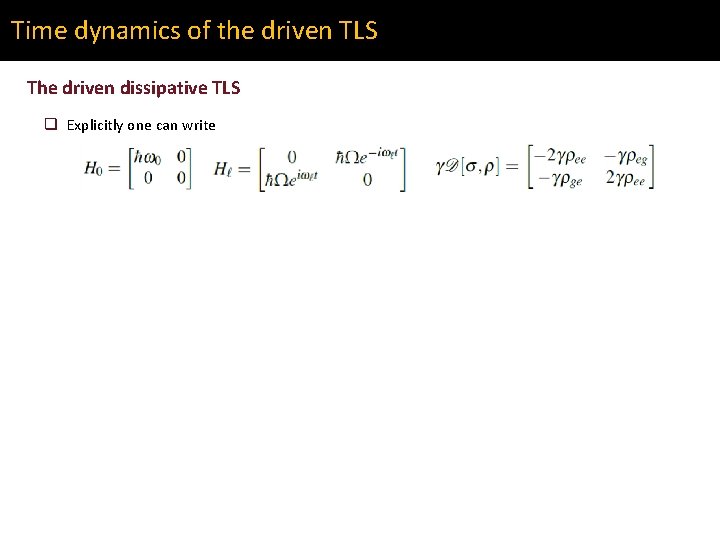
Time dynamics of the driven TLS The driven dissipative TLS q Explicitly one can write
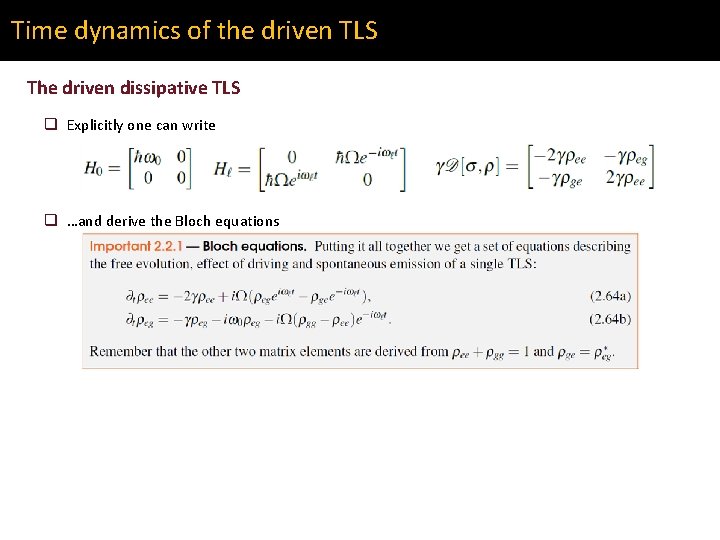
Time dynamics of the driven TLS The driven dissipative TLS q Explicitly one can write q …and derive the Bloch equations
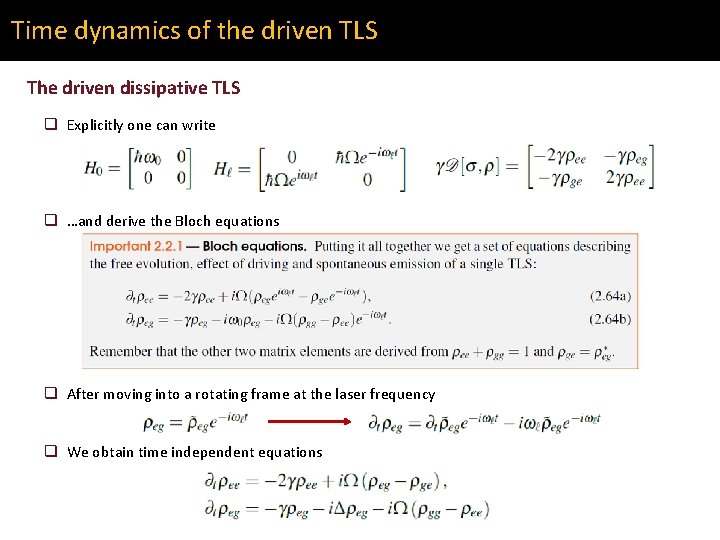
Time dynamics of the driven TLS The driven dissipative TLS q Explicitly one can write q …and derive the Bloch equations q After moving into a rotating frame at the laser frequency q We obtain time independent equations
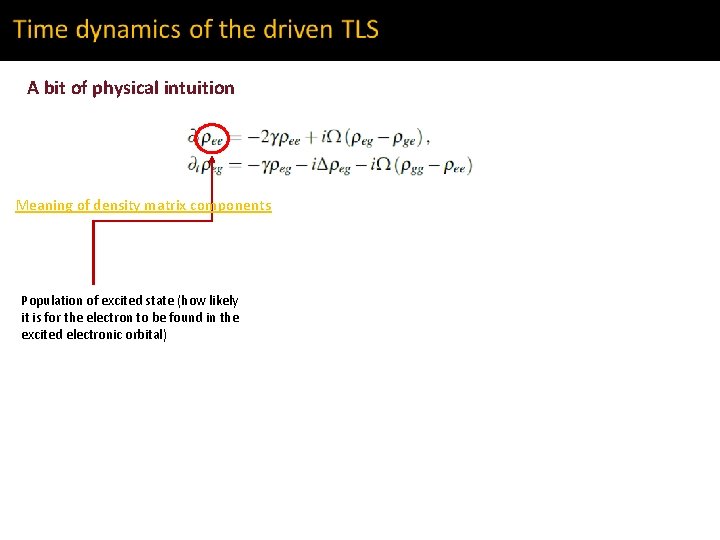
A bit of physical intuition Meaning of density matrix components Population of excited state (how likely it is for the electron to be found in the excited electronic orbital)
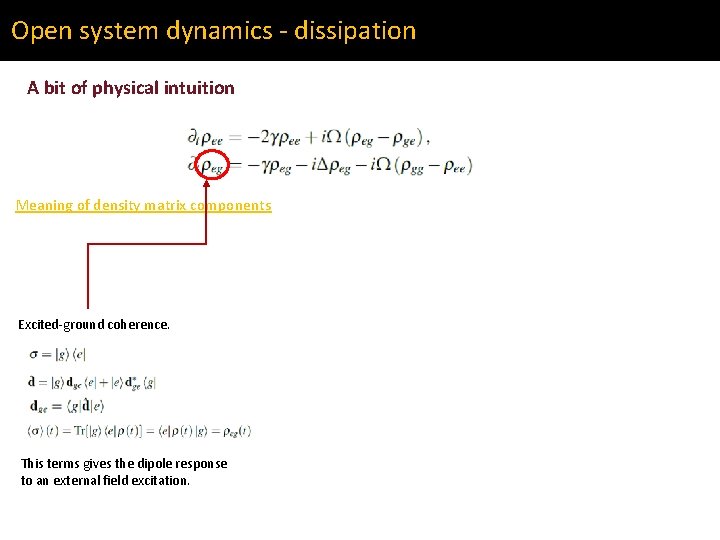
Open system dynamics - dissipation A bit of physical intuition Meaning of density matrix components Excited-ground coherence. This terms gives the dipole response to an external field excitation.
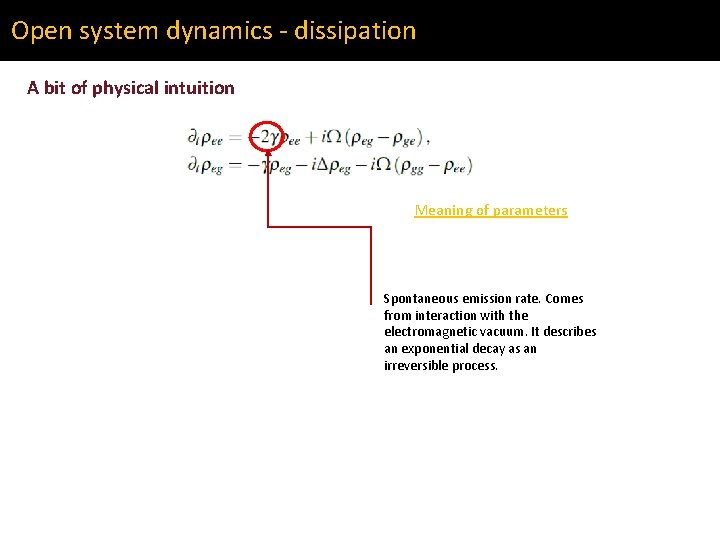
Open system dynamics - dissipation A bit of physical intuition Meaning of parameters Spontaneous emission rate. Comes from interaction with the electromagnetic vacuum. It describes an exponential decay as an irreversible process.
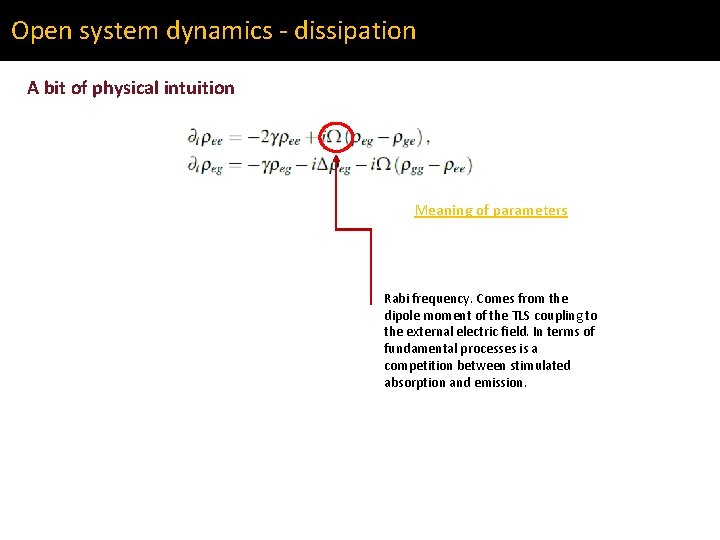
Open system dynamics - dissipation A bit of physical intuition Meaning of parameters Rabi frequency. Comes from the dipole moment of the TLS coupling to the external electric field. In terms of fundamental processes is a competition between stimulated absorption and emission.
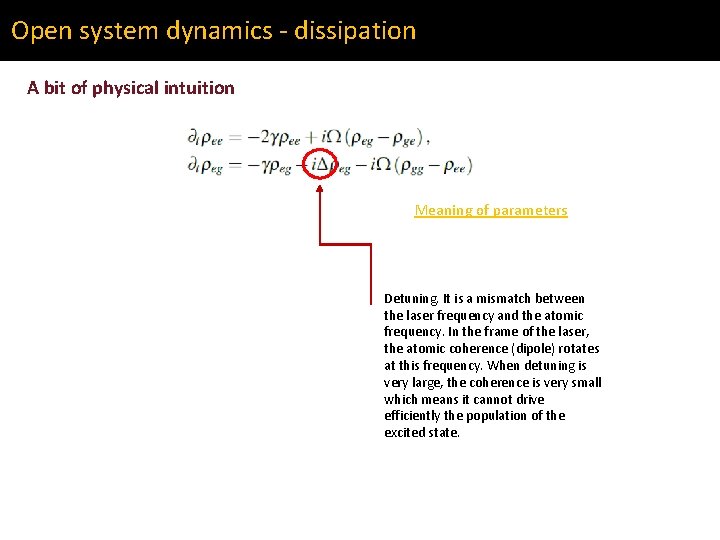
Open system dynamics - dissipation A bit of physical intuition Meaning of parameters Detuning. It is a mismatch between the laser frequency and the atomic frequency. In the frame of the laser, the atomic coherence (dipole) rotates at this frequency. When detuning is very large, the coherence is very small which means it cannot drive efficiently the population of the excited state.
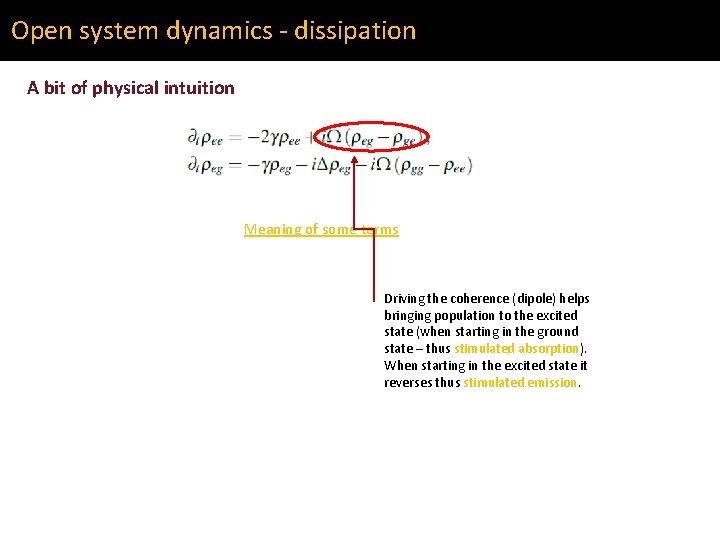
Open system dynamics - dissipation A bit of physical intuition Meaning of some terms Driving the coherence (dipole) helps bringing population to the excited state (when starting in the ground state – thus stimulated absorption). When starting in the excited state it reverses thus stimulated emission.
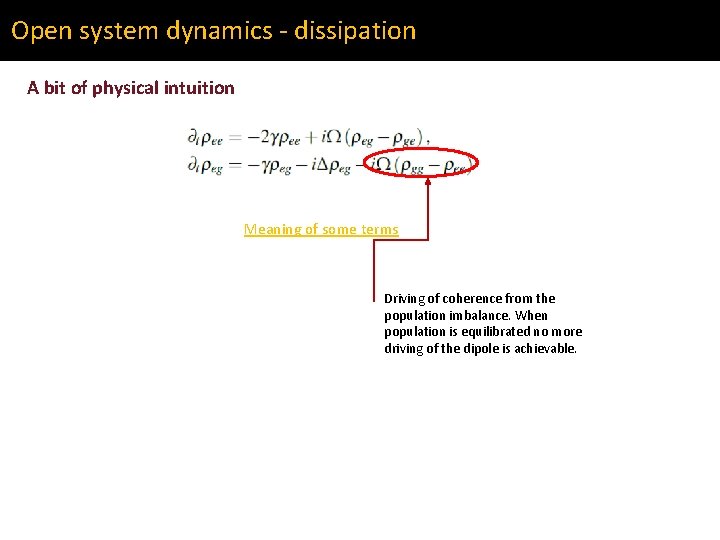
Open system dynamics - dissipation A bit of physical intuition Meaning of some terms Driving of coherence from the population imbalance. When population is equilibrated no more driving of the dipole is achievable.
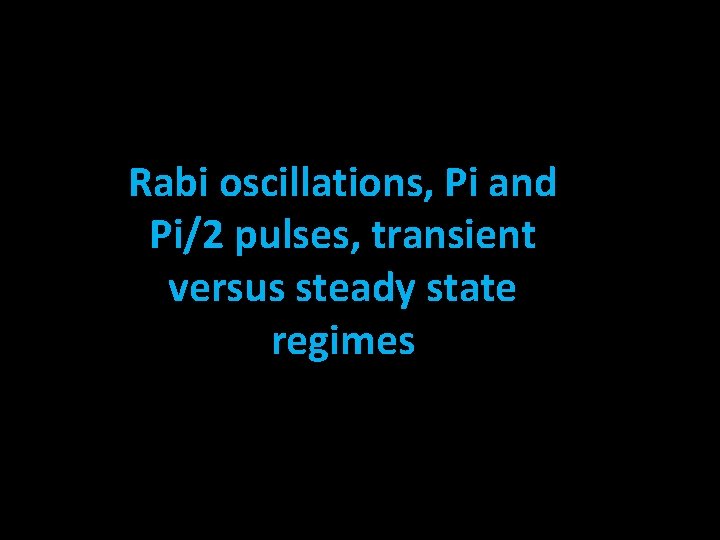
Rabi oscillations, Pi and Pi/2 pulses, transient versus steady state regimes
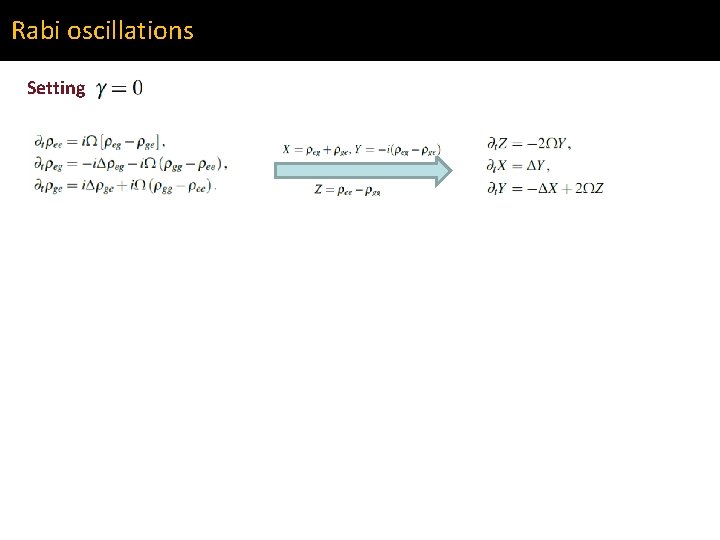
Rabi oscillations Setting
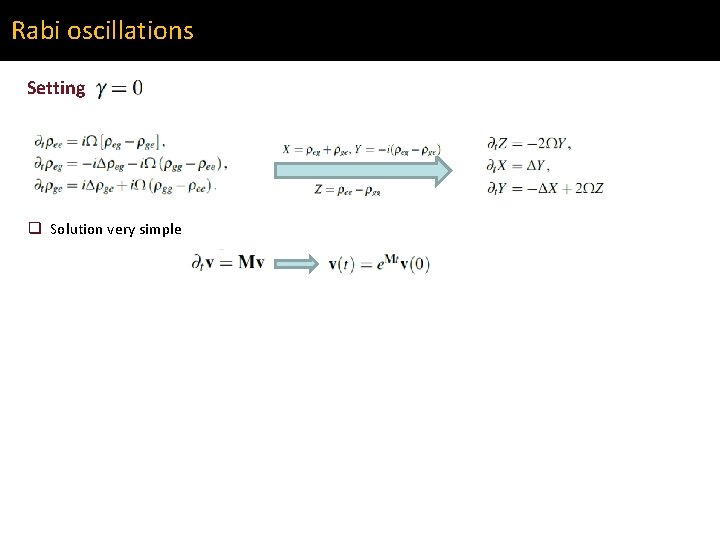
Rabi oscillations Setting q Solution very simple
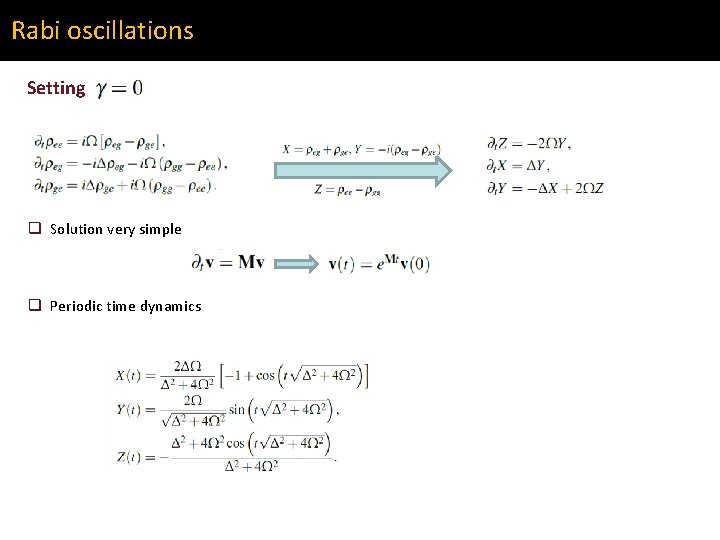
Rabi oscillations Setting q Solution very simple q Periodic time dynamics
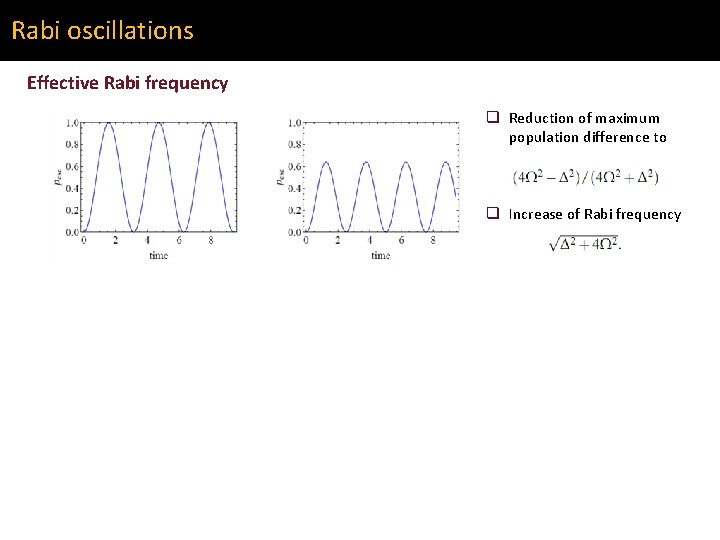
Rabi oscillations Effective Rabi frequency q Reduction of maximum population difference to q Increase of Rabi frequency
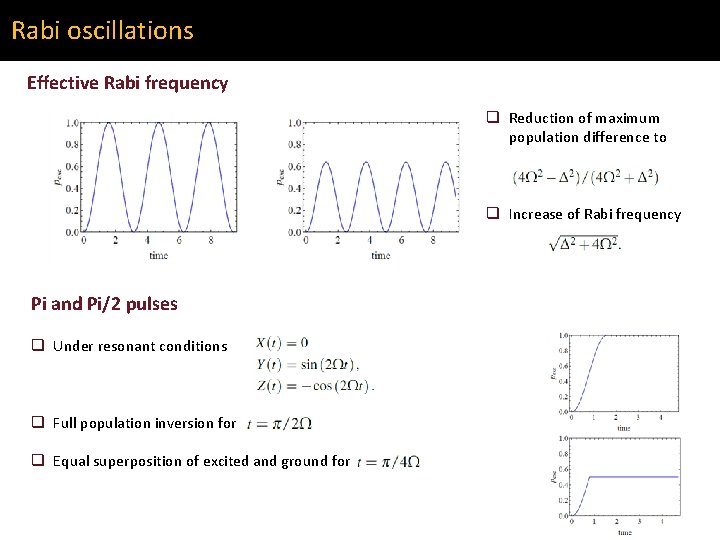
Rabi oscillations Effective Rabi frequency q Reduction of maximum population difference to q Increase of Rabi frequency Pi and Pi/2 pulses q Under resonant conditions q Full population inversion for q Equal superposition of excited and ground for
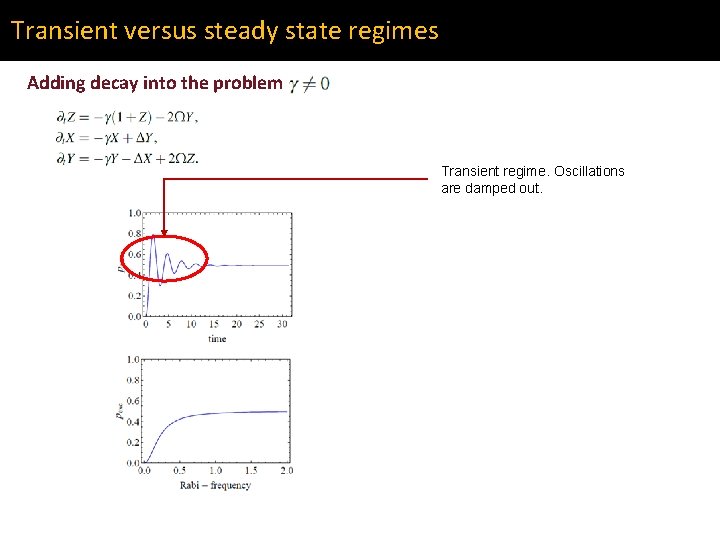
Transient versus steady state regimes Adding decay into the problem Transient regime. Oscillations are damped out.
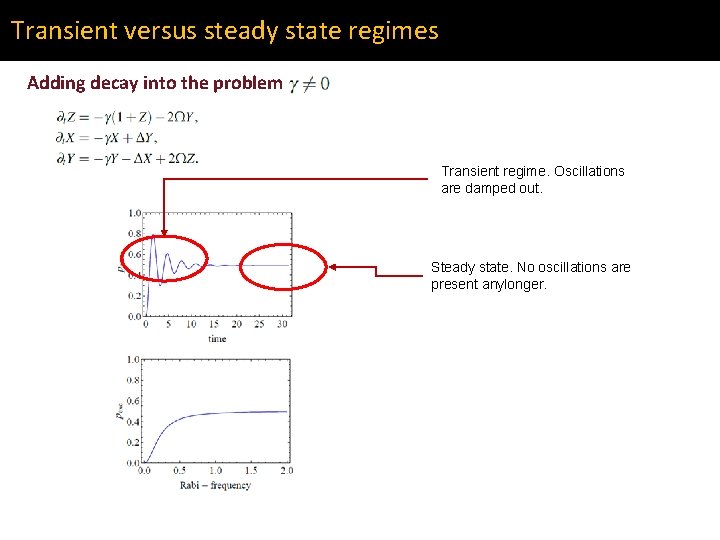
Transient versus steady state regimes Adding decay into the problem Transient regime. Oscillations are damped out. Steady state. No oscillations are present anylonger.
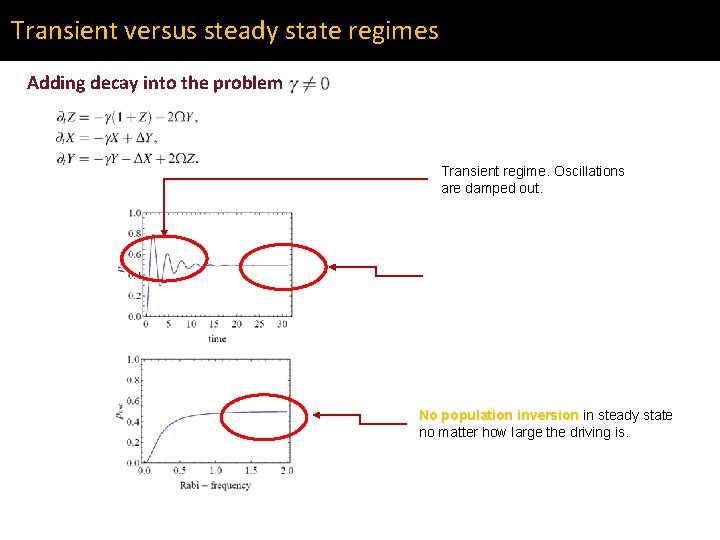
Transient versus steady state regimes Adding decay into the problem Transient regime. Oscillations are damped out. No population inversion in steady state no matter how large the driving is.
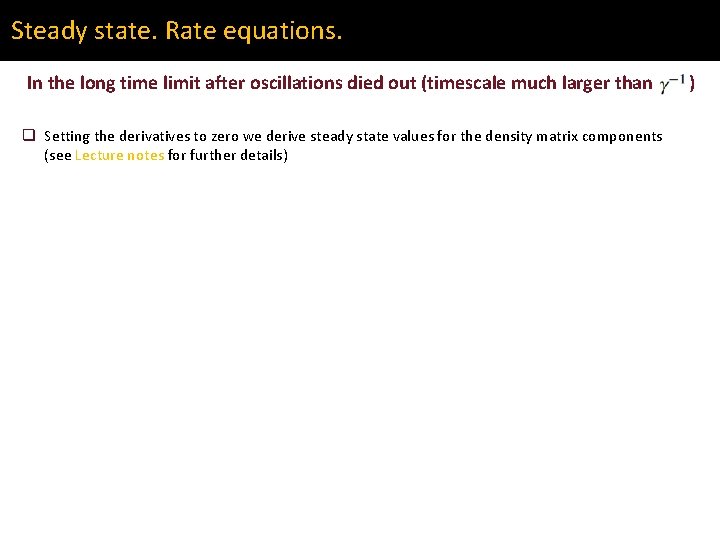
Steady state. Rate equations. In the long time limit after oscillations died out (timescale much larger than q Setting the derivatives to zero we derive steady state values for the density matrix components (see Lecture notes for further details) )
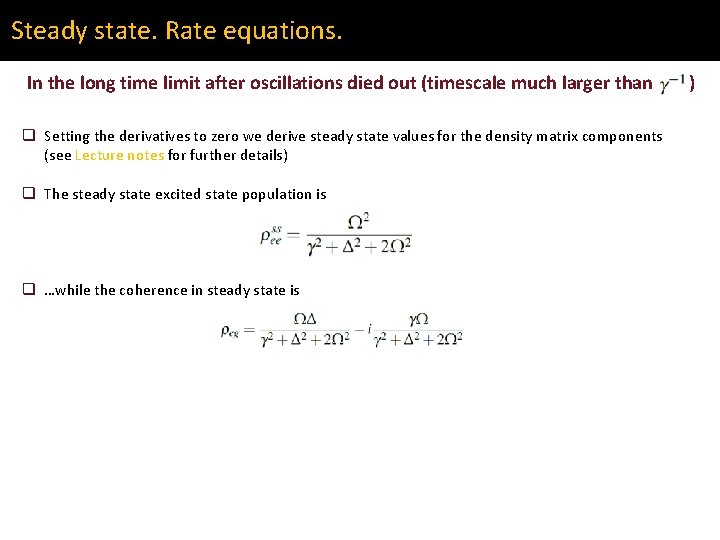
Steady state. Rate equations. In the long time limit after oscillations died out (timescale much larger than q Setting the derivatives to zero we derive steady state values for the density matrix components (see Lecture notes for further details) q The steady state excited state population is q …while the coherence in steady state is )
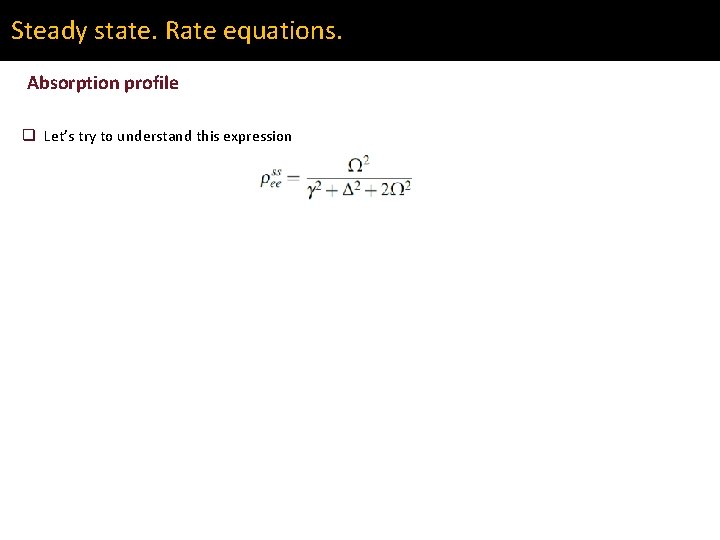
Steady state. Rate equations. Absorption profile q Let’s try to understand this expression
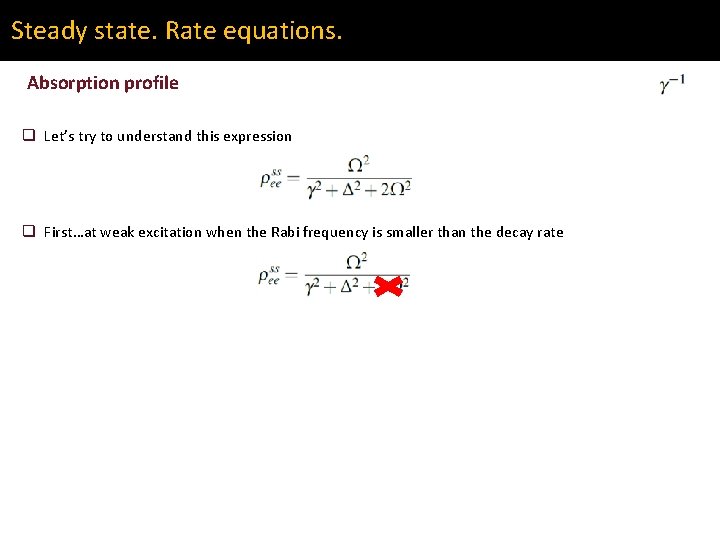
Steady state. Rate equations. Absorption profile q Let’s try to understand this expression q First…at weak excitation when the Rabi frequency is smaller than the decay rate
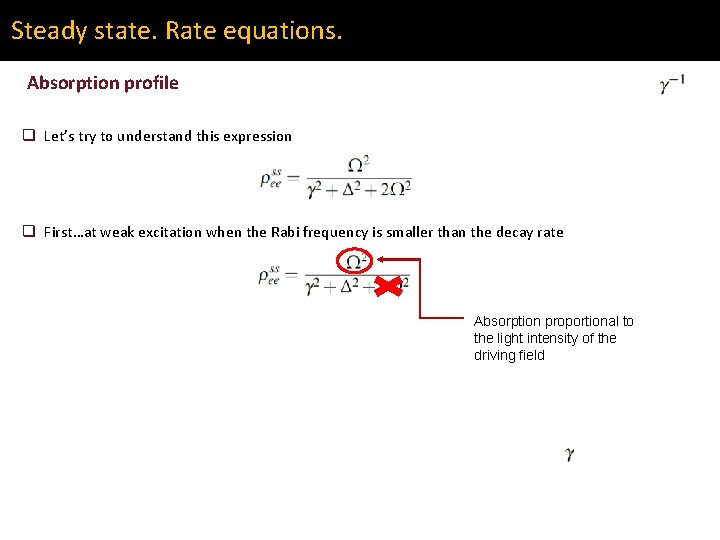
Steady state. Rate equations. Absorption profile q Let’s try to understand this expression q First…at weak excitation when the Rabi frequency is smaller than the decay rate Absorption proportional to the light intensity of the driving field
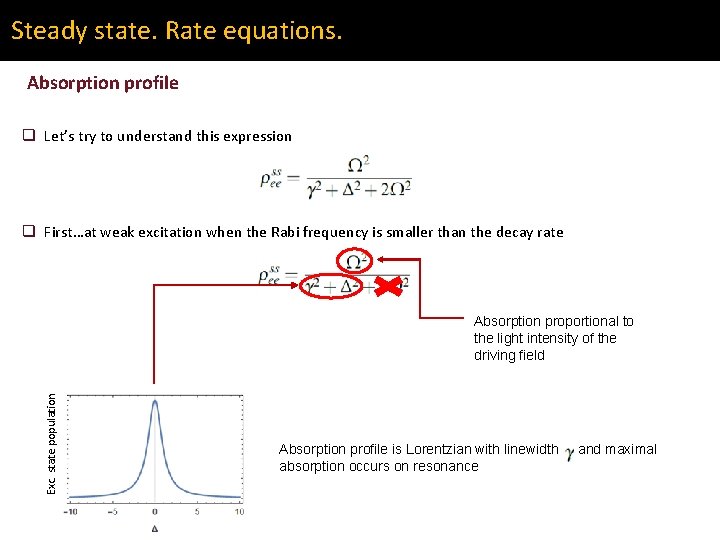
Steady state. Rate equations. Absorption profile q Let’s try to understand this expression q First…at weak excitation when the Rabi frequency is smaller than the decay rate Exc. state population Absorption proportional to the light intensity of the driving field Absorption profile is Lorentzian with linewidth absorption occurs on resonance and maximal
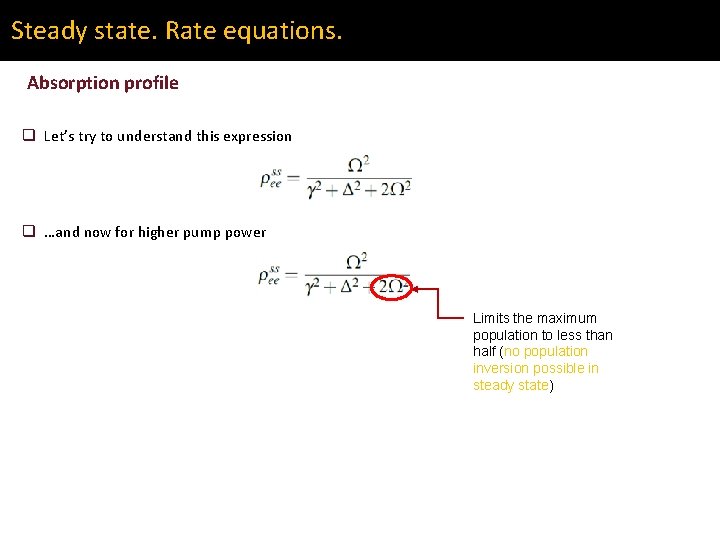
Steady state. Rate equations. Absorption profile q Let’s try to understand this expression q …and now for higher pump power Limits the maximum population to less than half (no population inversion possible in steady state)
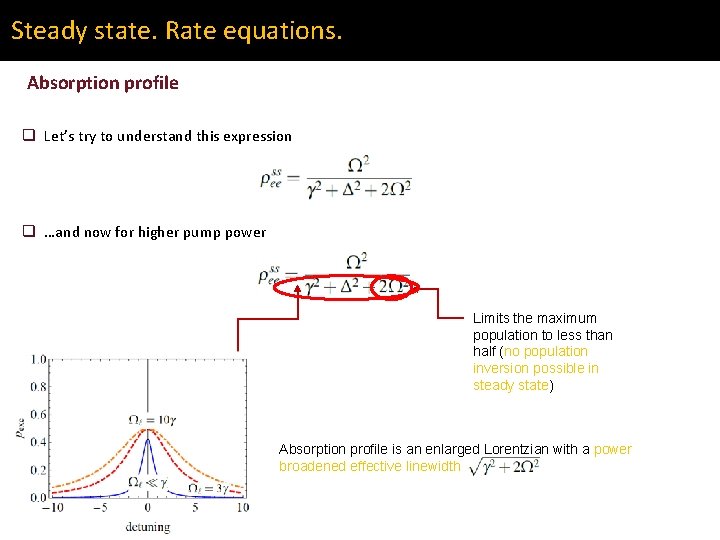
Steady state. Rate equations. Absorption profile q Let’s try to understand this expression q …and now for higher pump power Limits the maximum population to less than half (no population inversion possible in steady state) Absorption profile is an enlarged Lorentzian with a power broadened effective linewidth
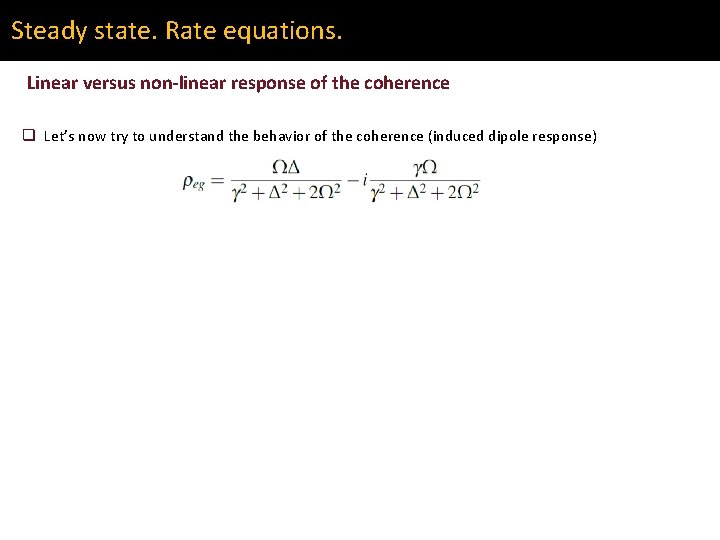
Steady state. Rate equations. Linear versus non-linear response of the coherence q Let’s now try to understand the behavior of the coherence (induced dipole response)
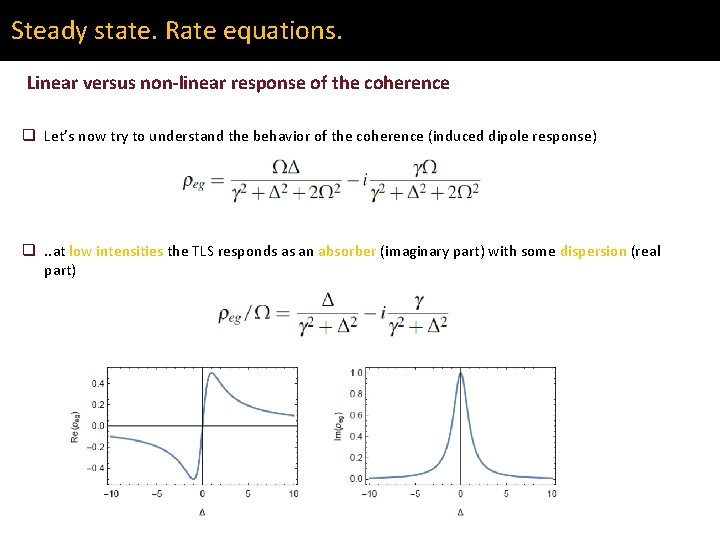
Steady state. Rate equations. Linear versus non-linear response of the coherence q Let’s now try to understand the behavior of the coherence (induced dipole response) q. . at low intensities the TLS responds as an absorber (imaginary part) with some dispersion (real part)
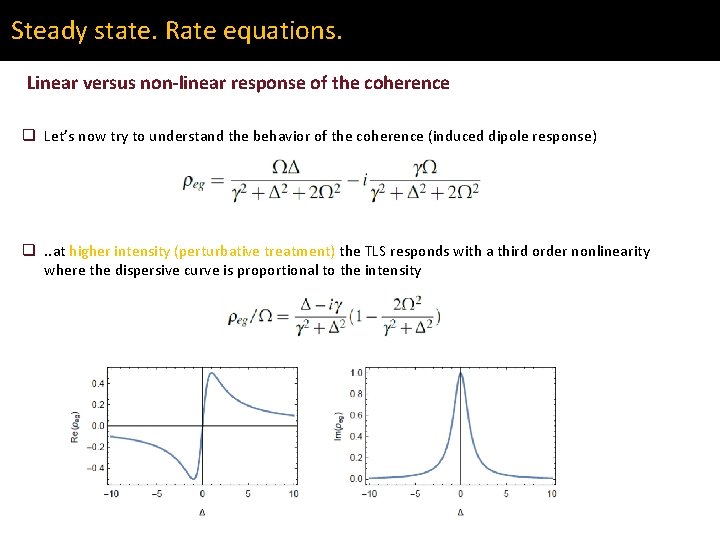
Steady state. Rate equations. Linear versus non-linear response of the coherence q Let’s now try to understand the behavior of the coherence (induced dipole response) q. . at higher intensity (perturbative treatment) the TLS responds with a third order nonlinearity where the dispersive curve is proportional to the intensity
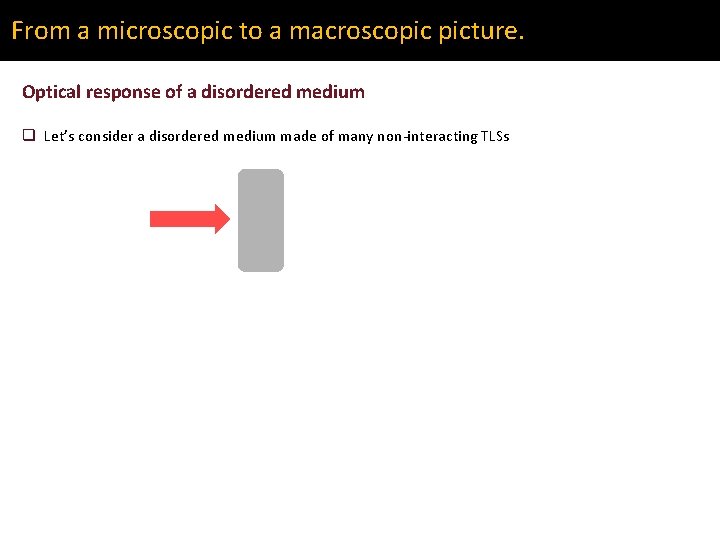
From a microscopic to a macroscopic picture. Optical response of a disordered medium q Let’s consider a disordered medium made of many non-interacting TLSs
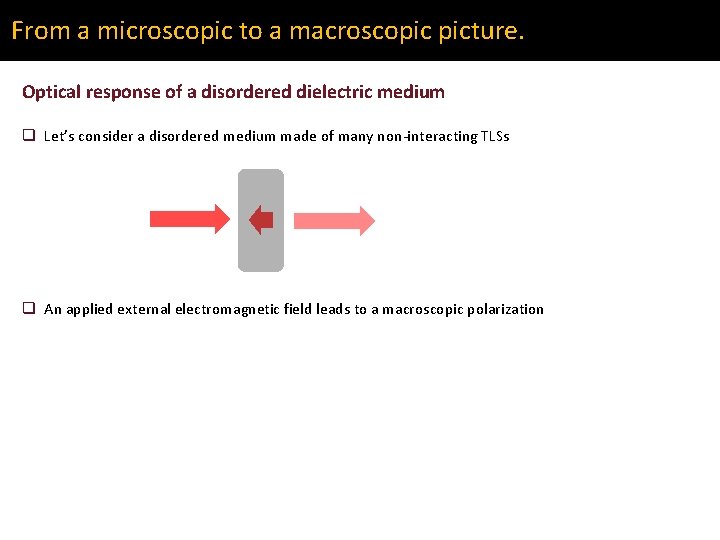
From a microscopic to a macroscopic picture. Optical response of a disordered dielectric medium q Let’s consider a disordered medium made of many non-interacting TLSs q An applied external electromagnetic field leads to a macroscopic polarization
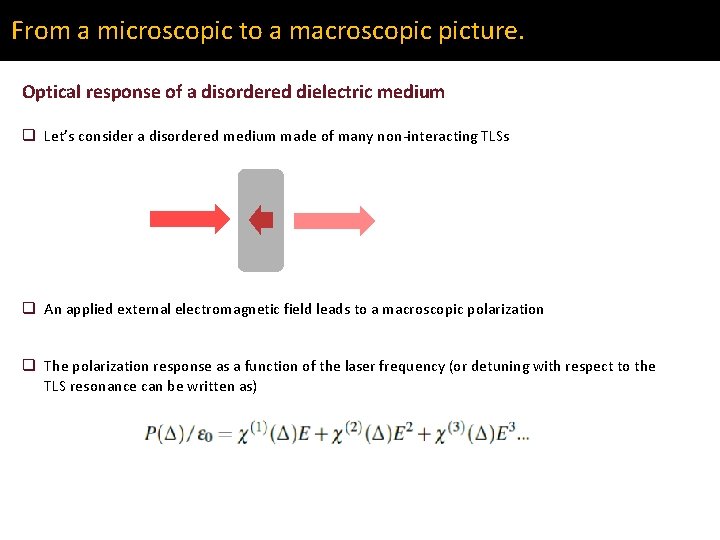
From a microscopic to a macroscopic picture. Optical response of a disordered dielectric medium q Let’s consider a disordered medium made of many non-interacting TLSs q An applied external electromagnetic field leads to a macroscopic polarization q The polarization response as a function of the laser frequency (or detuning with respect to the TLS resonance can be written as)
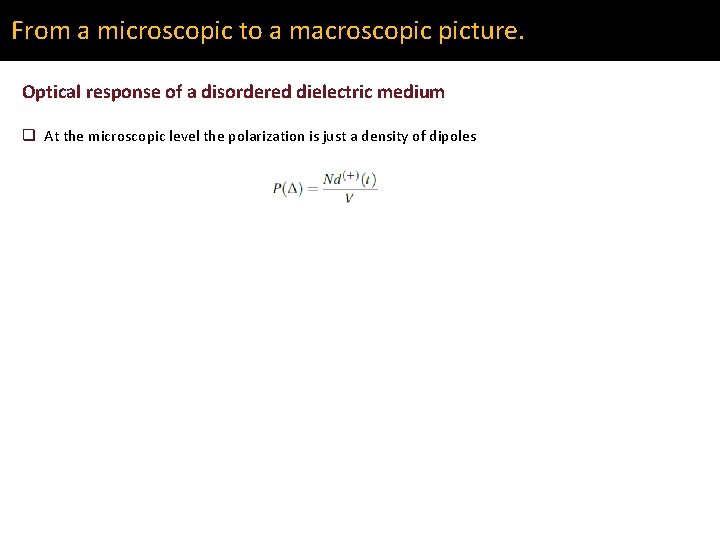
From a microscopic to a macroscopic picture. Optical response of a disordered dielectric medium q At the microscopic level the polarization is just a density of dipoles
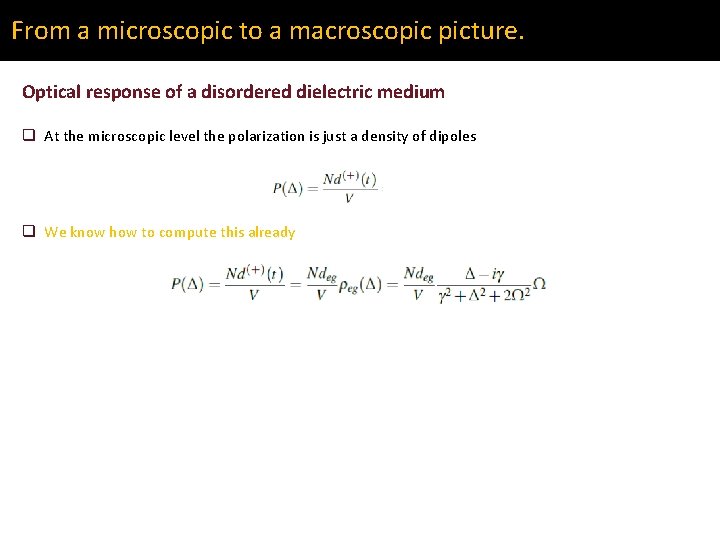
From a microscopic to a macroscopic picture. Optical response of a disordered dielectric medium q At the microscopic level the polarization is just a density of dipoles q We know how to compute this already
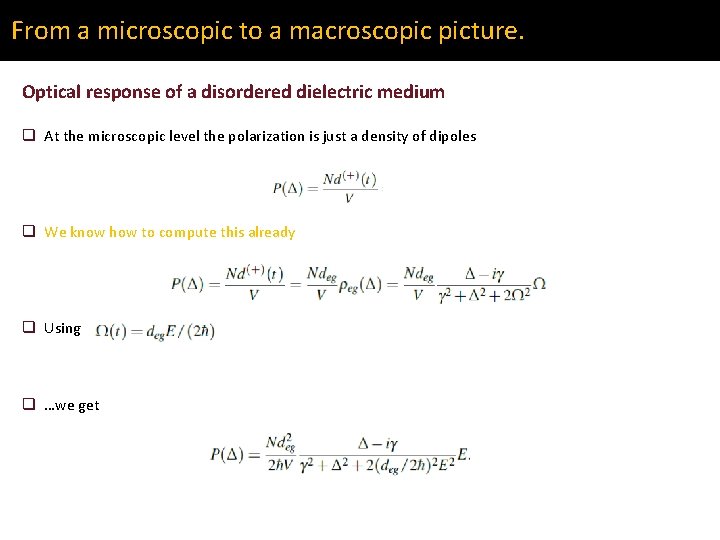
From a microscopic to a macroscopic picture. Optical response of a disordered dielectric medium q At the microscopic level the polarization is just a density of dipoles q We know how to compute this already q Using q …we get
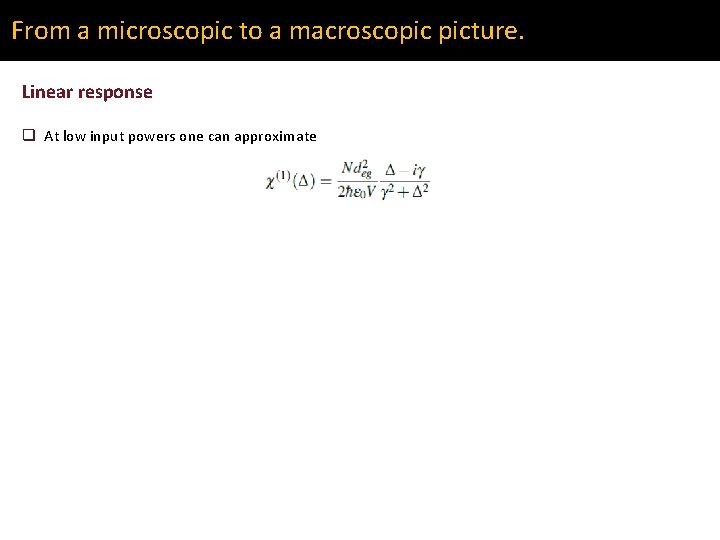
From a microscopic to a macroscopic picture. Linear response q At low input powers one can approximate
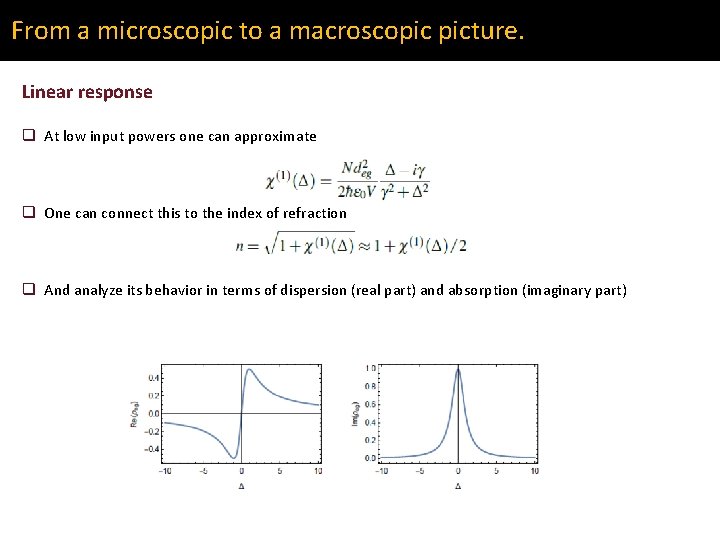
From a microscopic to a macroscopic picture. Linear response q At low input powers one can approximate q One can connect this to the index of refraction q And analyze its behavior in terms of dispersion (real part) and absorption (imaginary part)
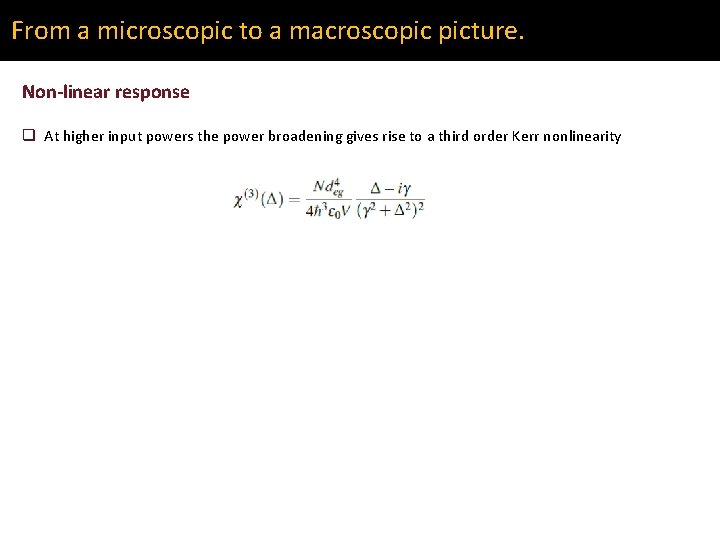
From a microscopic to a macroscopic picture. Non-linear response q At higher input powers the power broadening gives rise to a third order Kerr nonlinearity
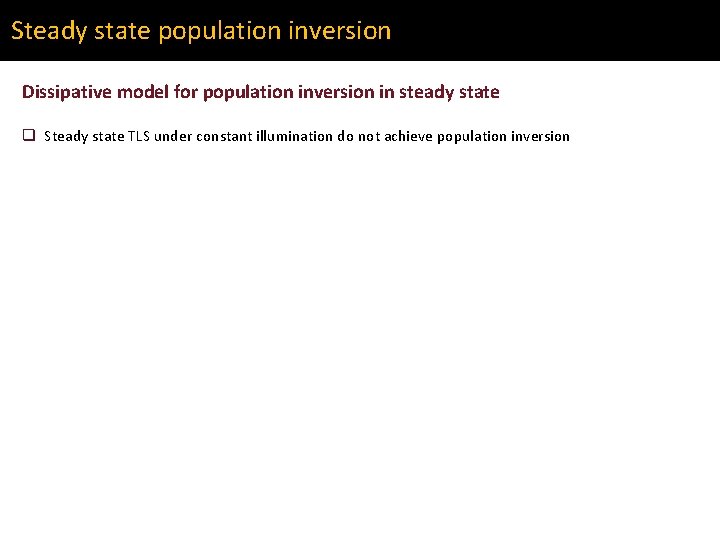
Steady state population inversion Dissipative model for population inversion in steady state q Steady state TLS under constant illumination do not achieve population inversion
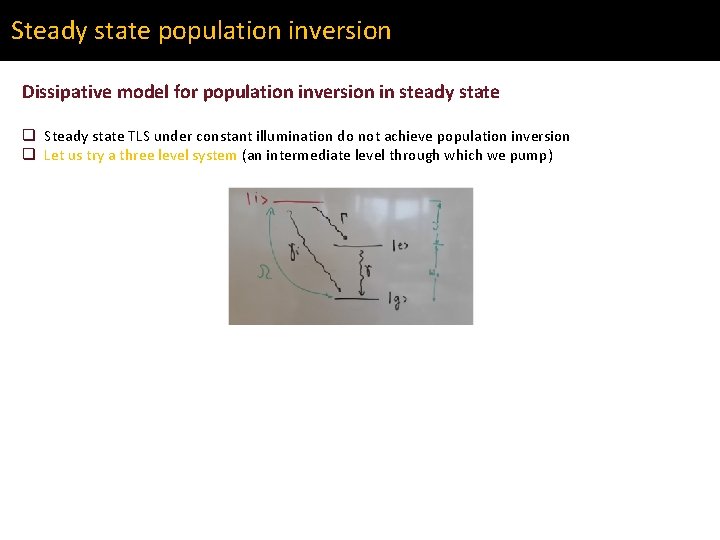
Steady state population inversion Dissipative model for population inversion in steady state q Steady state TLS under constant illumination do not achieve population inversion q Let us try a three level system (an intermediate level through which we pump)
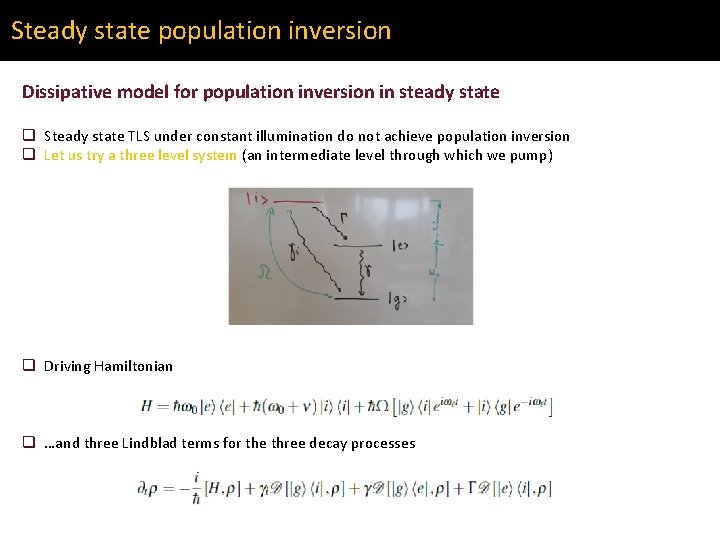
Steady state population inversion Dissipative model for population inversion in steady state q Steady state TLS under constant illumination do not achieve population inversion q Let us try a three level system (an intermediate level through which we pump) q Driving Hamiltonian q …and three Lindblad terms for the three decay processes
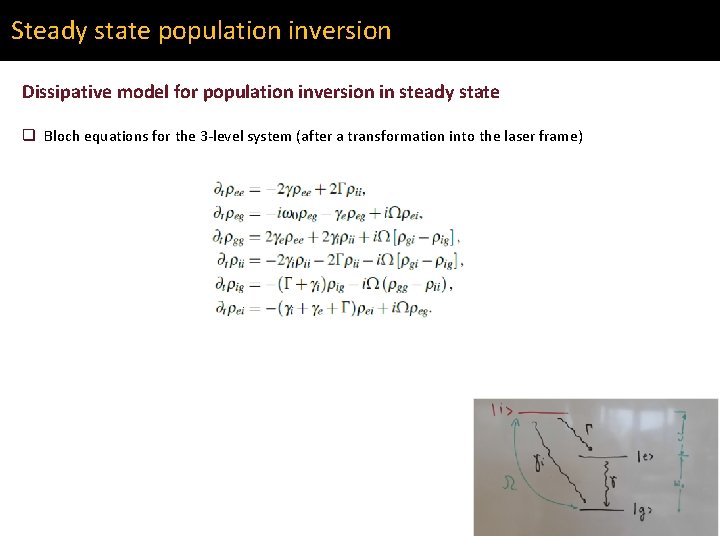
Steady state population inversion Dissipative model for population inversion in steady state q Bloch equations for the 3 -level system (after a transformation into the laser frame)
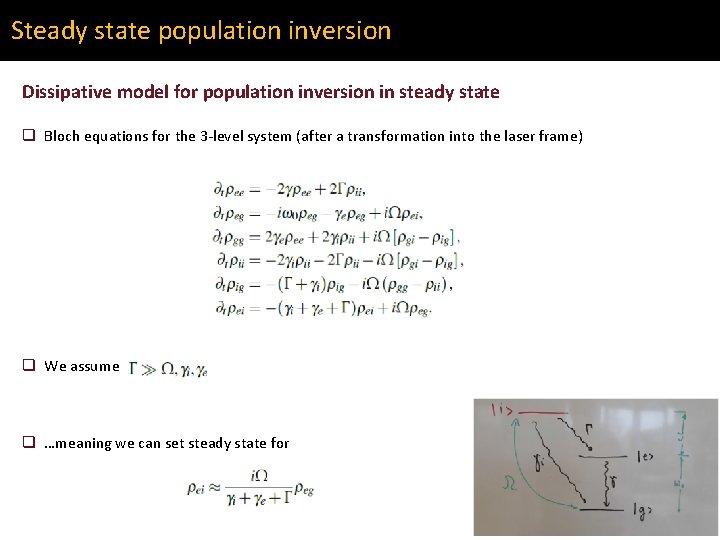
Steady state population inversion Dissipative model for population inversion in steady state q Bloch equations for the 3 -level system (after a transformation into the laser frame) q We assume q …meaning we can set steady state for
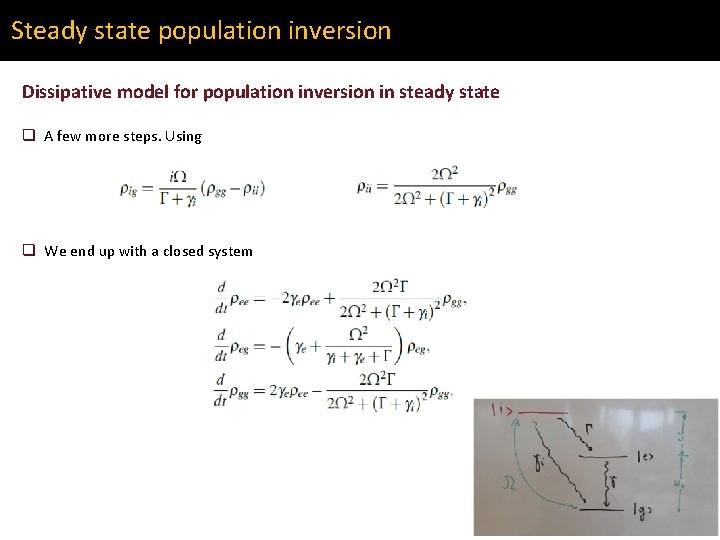
Steady state population inversion Dissipative model for population inversion in steady state q A few more steps. Using q We end up with a closed system
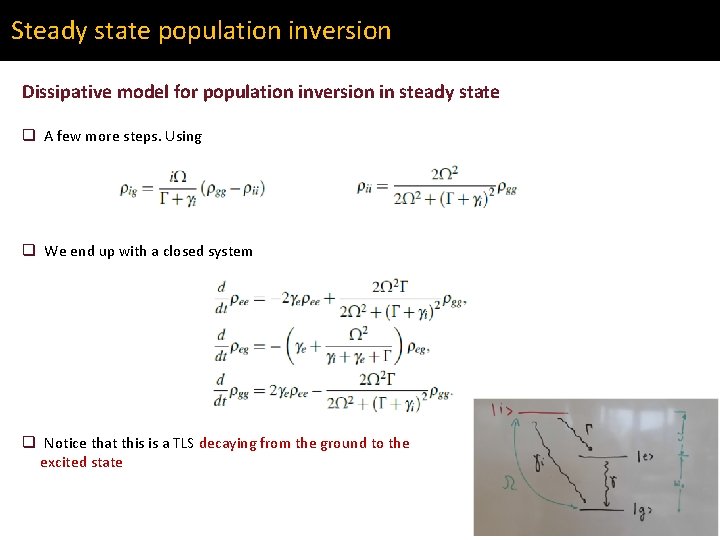
Steady state population inversion Dissipative model for population inversion in steady state q A few more steps. Using q We end up with a closed system q Notice that this is a TLS decaying from the ground to the excited state
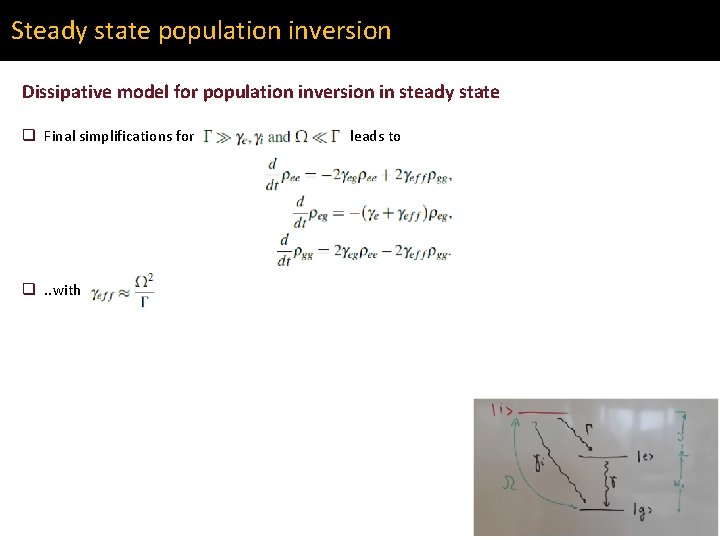
Steady state population inversion Dissipative model for population inversion in steady state q Final simplifications for q. . with leads to
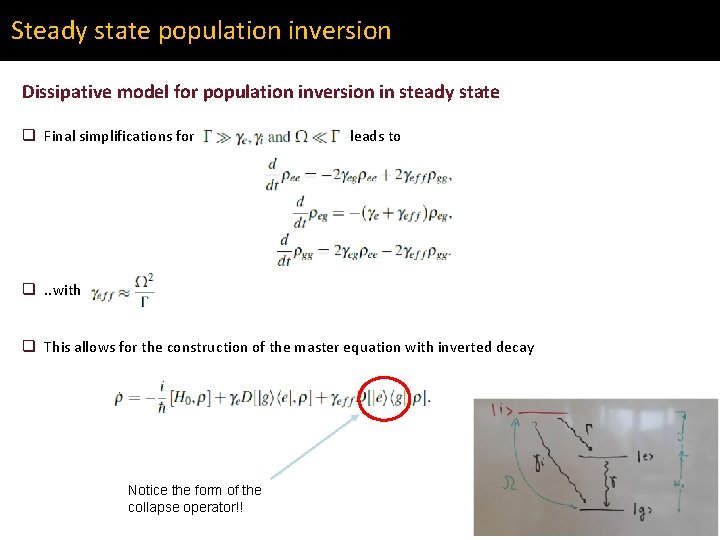
Steady state population inversion Dissipative model for population inversion in steady state q Final simplifications for leads to q. . with q This allows for the construction of the master equation with inverted decay Notice the form of the collapse operator!!
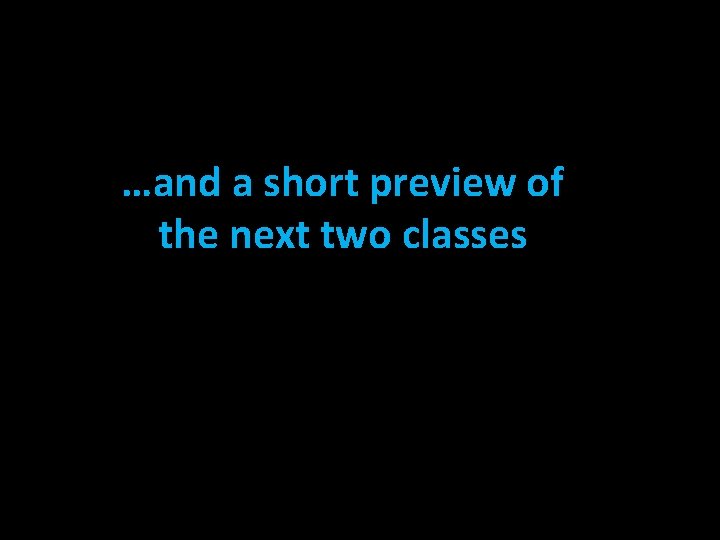
…and a short preview of the next two classes
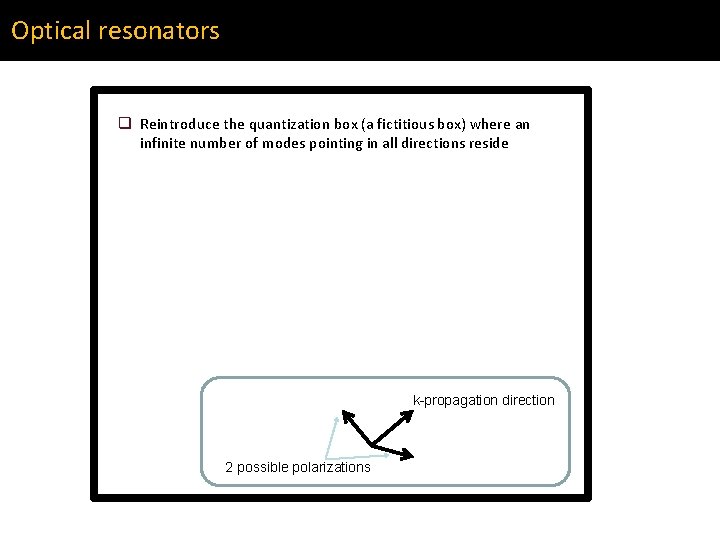
Optical resonators q Reintroduce the quantization box (a fictitious box) where an infinite number of modes pointing in all directions reside k-propagation direction 2 possible polarizations
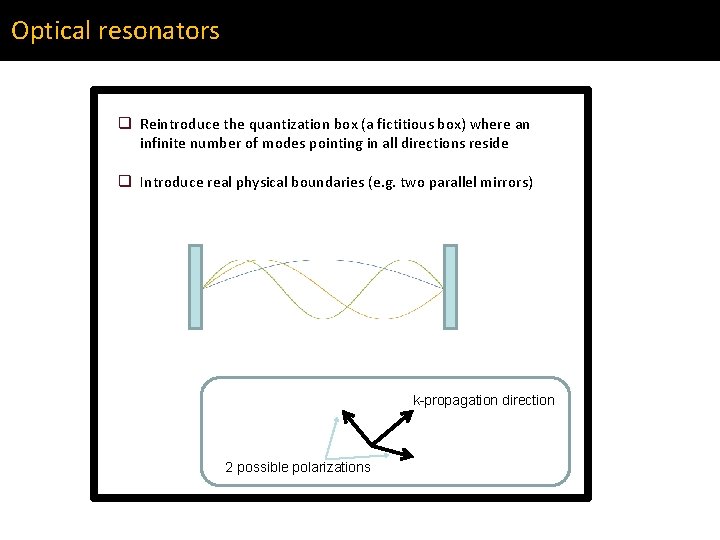
Optical resonators q Reintroduce the quantization box (a fictitious box) where an infinite number of modes pointing in all directions reside q Introduce real physical boundaries (e. g. two parallel mirrors) k-propagation direction 2 possible polarizations
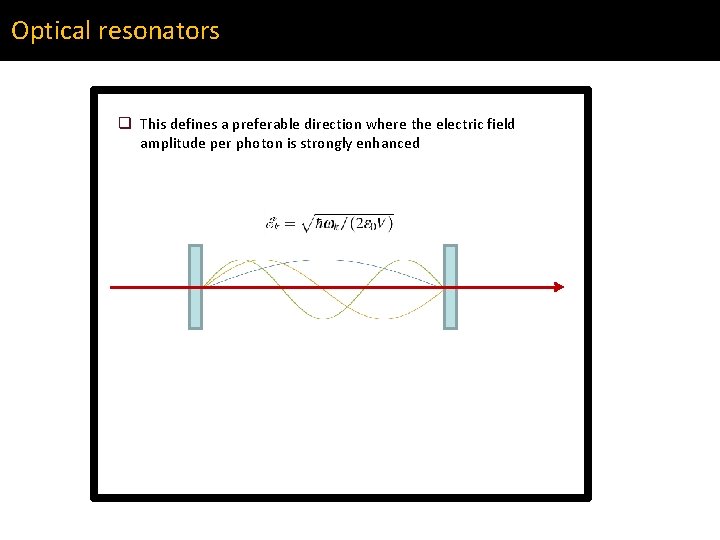
Optical resonators q This defines a preferable direction where the electric field amplitude per photon is strongly enhanced
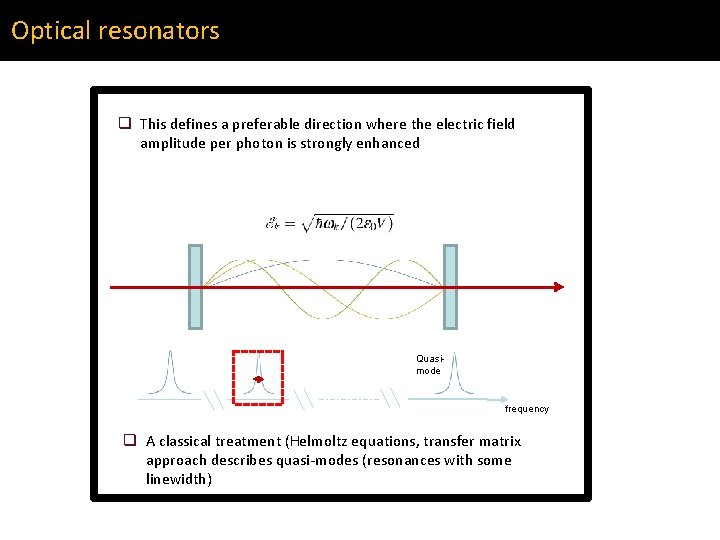
Optical resonators q This defines a preferable direction where the electric field amplitude per photon is strongly enhanced Quasimode frequency q A classical treatment (Helmoltz equations, transfer matrix approach describes quasi-modes (resonances with some linewidth)
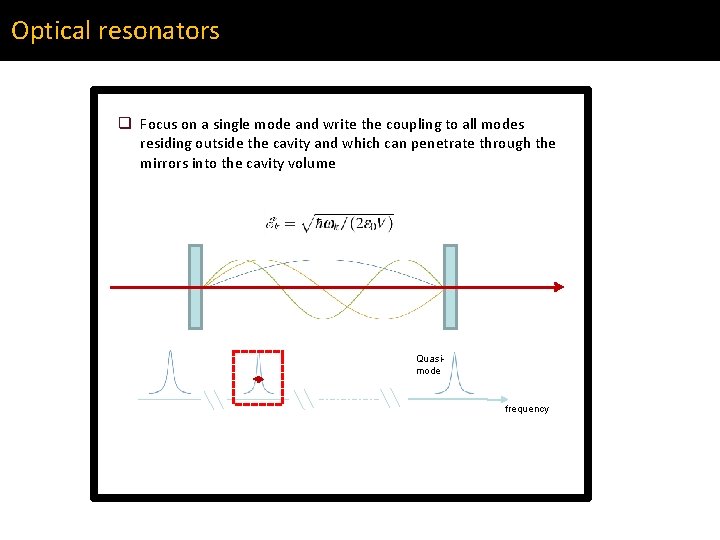
Optical resonators q Focus on a single mode and write the coupling to all modes residing outside the cavity and which can penetrate through the mirrors into the cavity volume Quasimode frequency
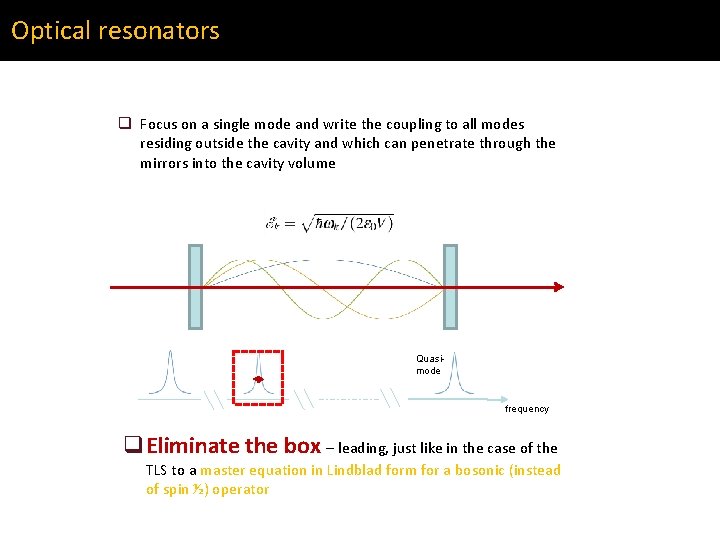
Optical resonators q Focus on a single mode and write the coupling to all modes residing outside the cavity and which can penetrate through the mirrors into the cavity volume Quasimode frequency q. Eliminate the box – leading, just like in the case of the TLS to a master equation in Lindblad form for a bosonic (instead of spin ½) operator
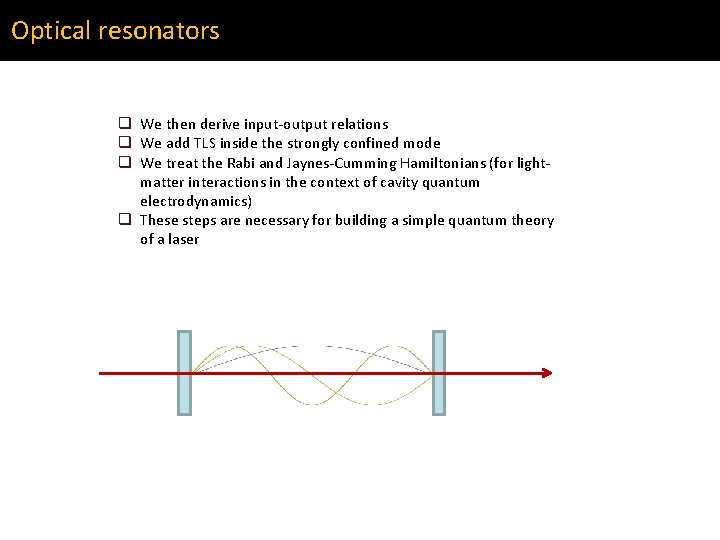
Optical resonators q We then derive input-output relations q We add TLS inside the strongly confined mode q We treat the Rabi and Jaynes-Cumming Hamiltonians (for lightmatter interactions in the context of cavity quantum electrodynamics) q These steps are necessary for building a simple quantum theory of a laser
Quantum physics vs mechanics
Quantum physics vs mechanics
Time dependent schrodinger wave equation
Quantum physics in hindi
Electromagnetism khan academy
Physics topic 12
Ap physics quantum mechanics
Seth aubin
Quantum and nuclear physics
Quantum physics wave function
Quantum physics summary
Quantum physics wave function
Hl physics ia ideas
Modern physics vs classical physics
University physics with modern physics fifteenth edition
Noncovalent interactions
Niches and community interactions
Special interactions
Chapter 22 reaching out cross-cultural interactions
Communication factors
Epistasis types
Parasitism
Dna-protein interactions
Geosphere examples
Intramolecular interactions
Protein binding interactions
Qualitative vs quantitative variable
Hccee
Chapter 22 reaching out cross-cultural interactions
Interactions among living things
Integral vs modular architecture
Integumentary interactions with other systems
Grapefruit-drug interactions chart
Fundamental particles and interactions
Congress formal and informal powers
Interactions
Cholinomimetic
Accounting information system chapter 1
Symbiosis and species interactions keystone webquest
Regional and transregional interactions
Ppi omeprazole
Opaque materials
Section 20-1 review species interactions
Sertraline interactions
Protein binding interactions
Reflection wave interactions
Nutrient interactions
Active form of vitamin e
Nervous interactions with other systems
Axial substituents
5 major types of species interactions
Interactions in the environment grade 7
Interactions between atoms occur
Parasitism
Protein binding interactions
Chapter 14 interactions in ecosystems
Functions of the digestive system
Brain warmer
Types of interactions
Interaction planning process
Macroeconomics unit 5 lesson 2 activity 45