Quantum Physics of LightMatter Interactions So Se 20
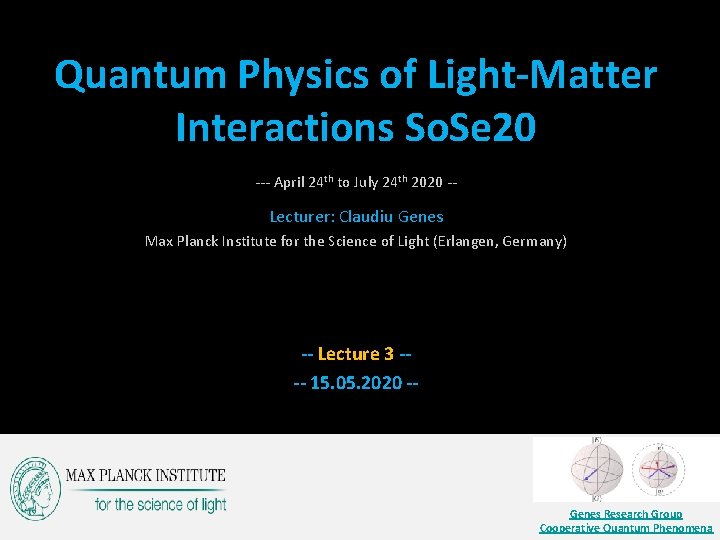
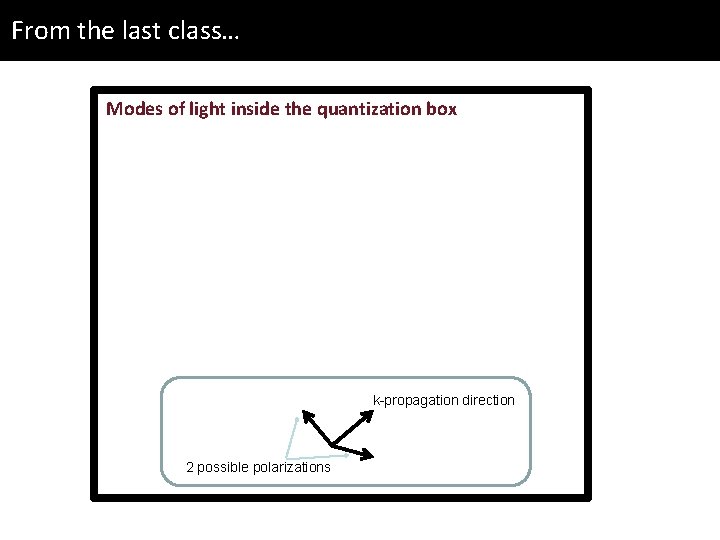
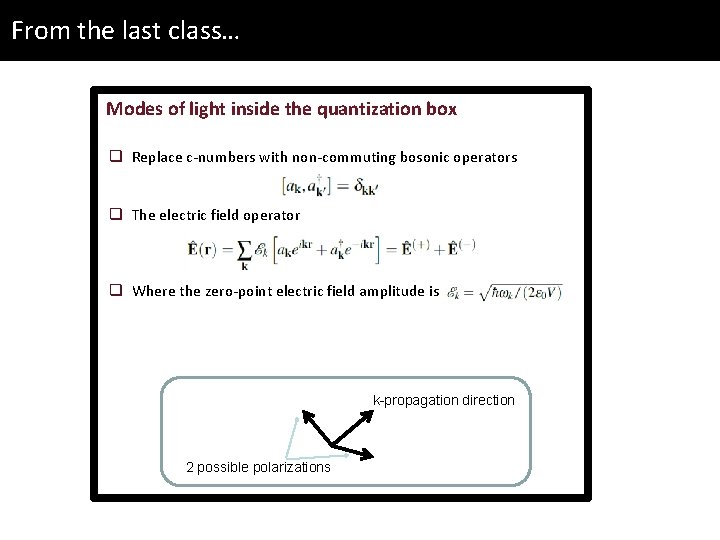
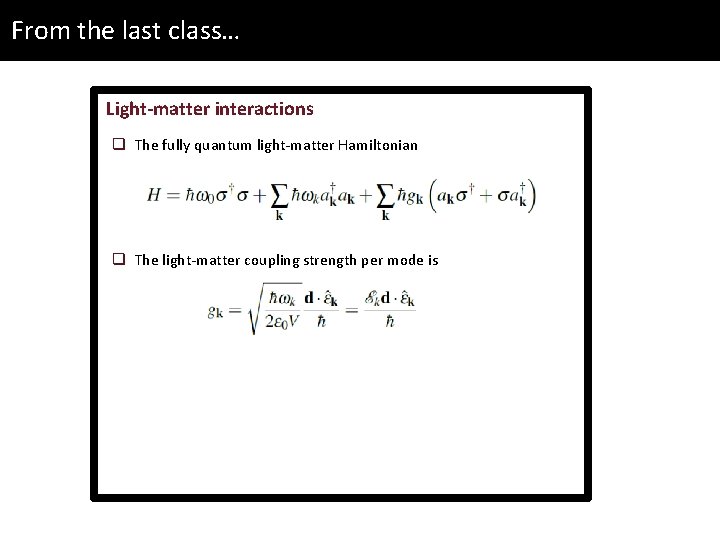
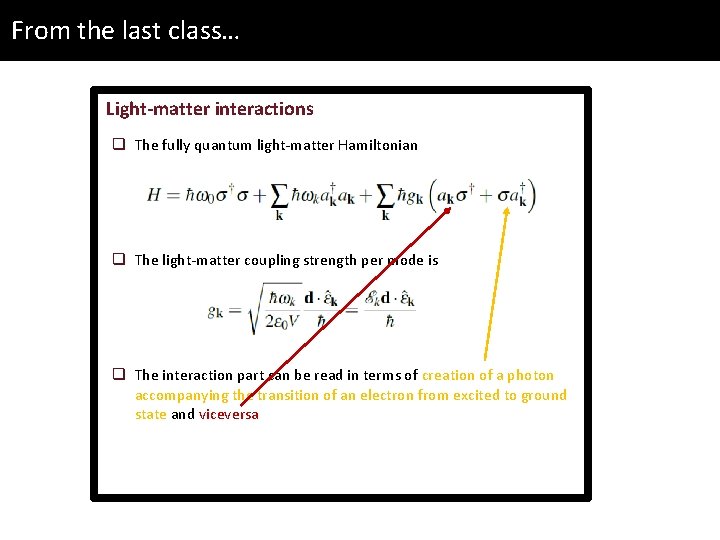
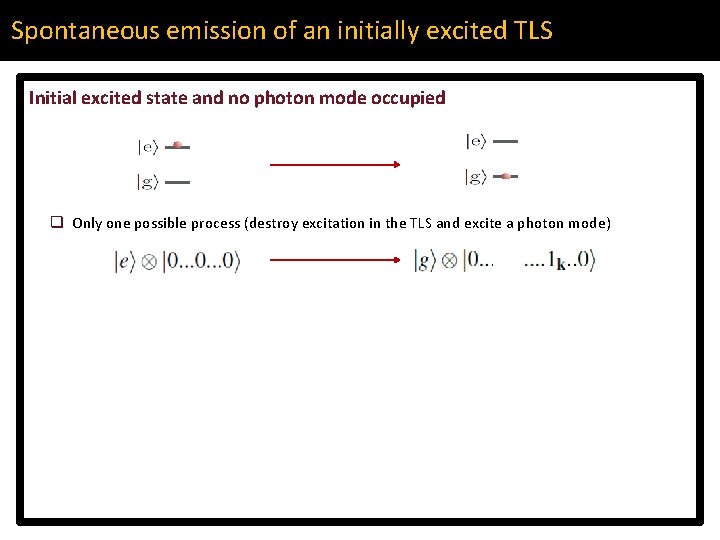
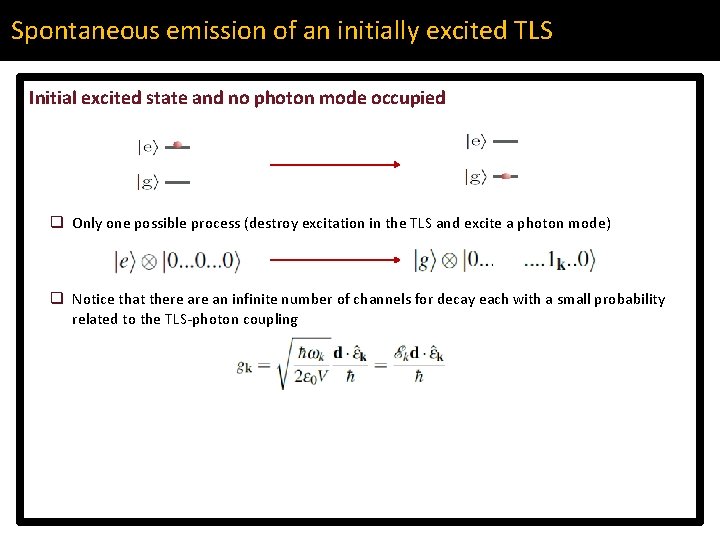
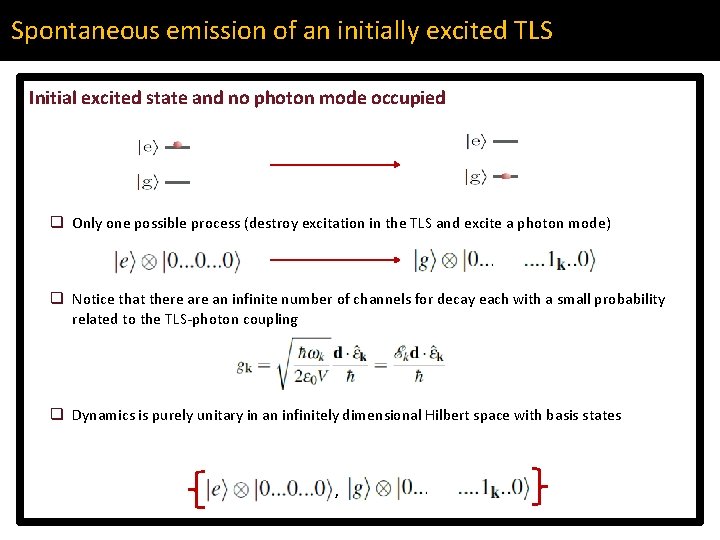
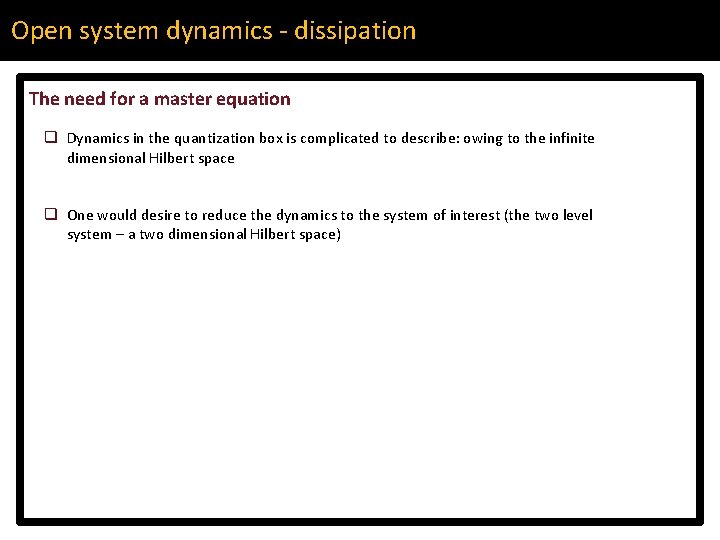
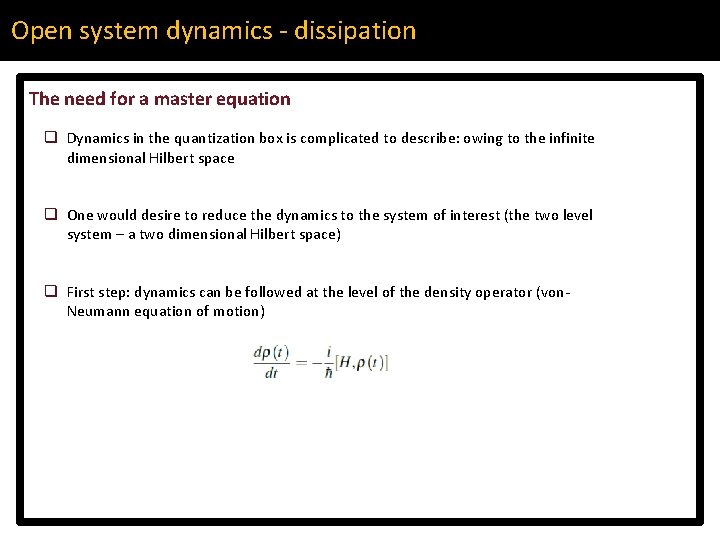
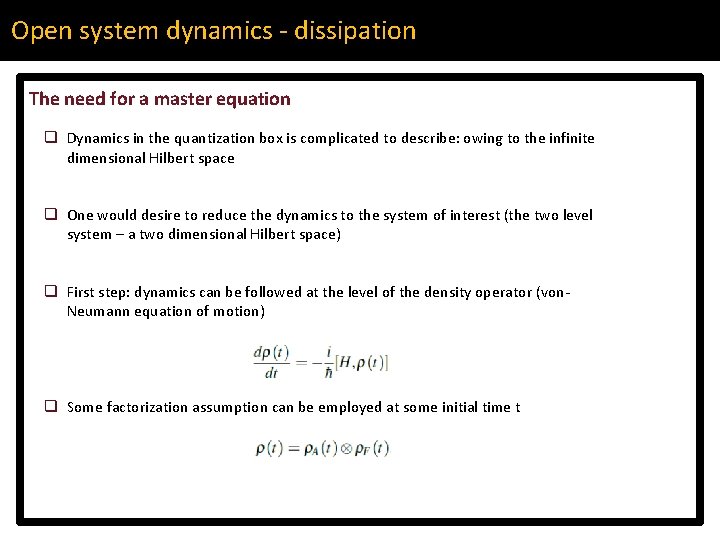
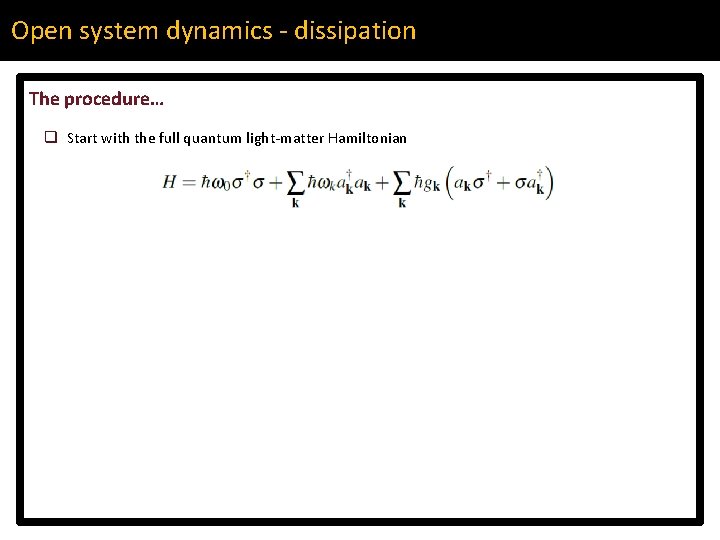
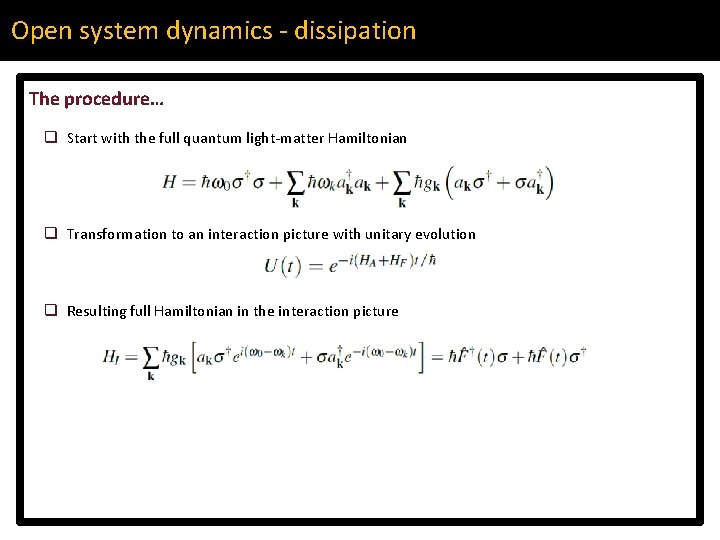
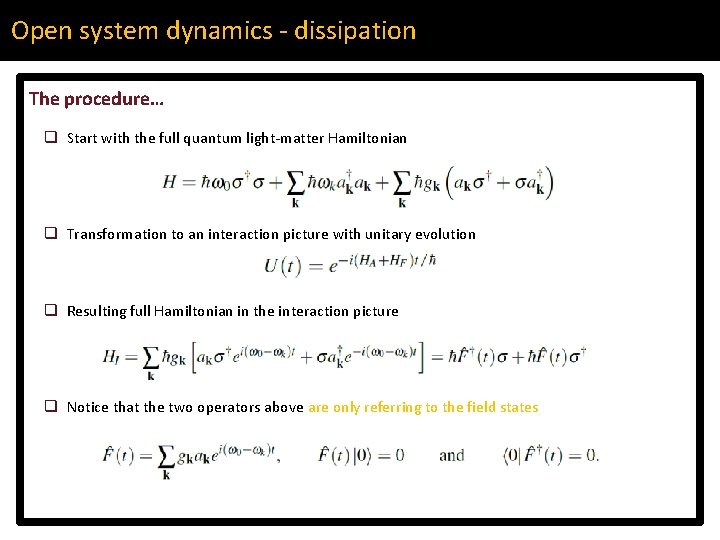
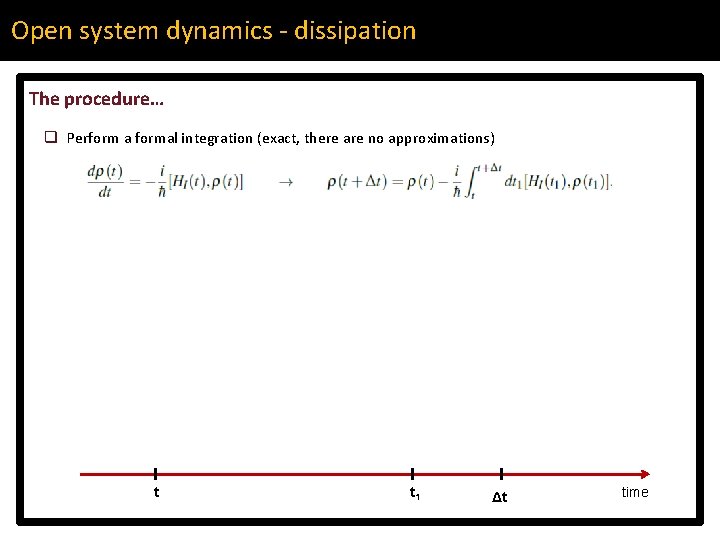
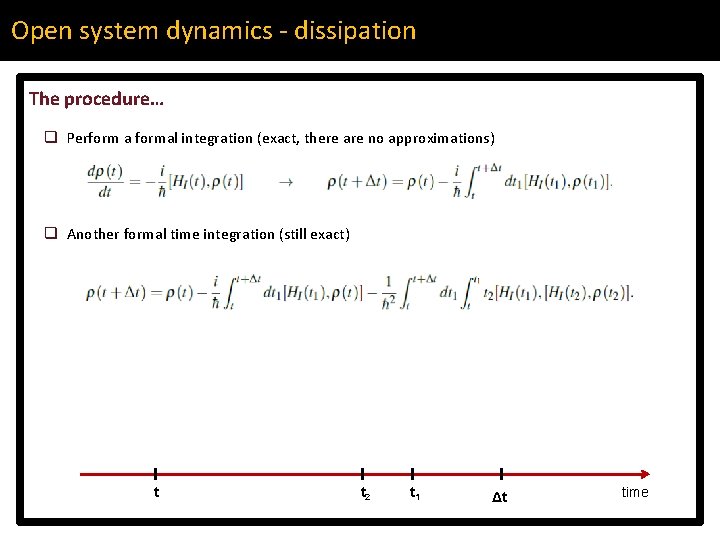
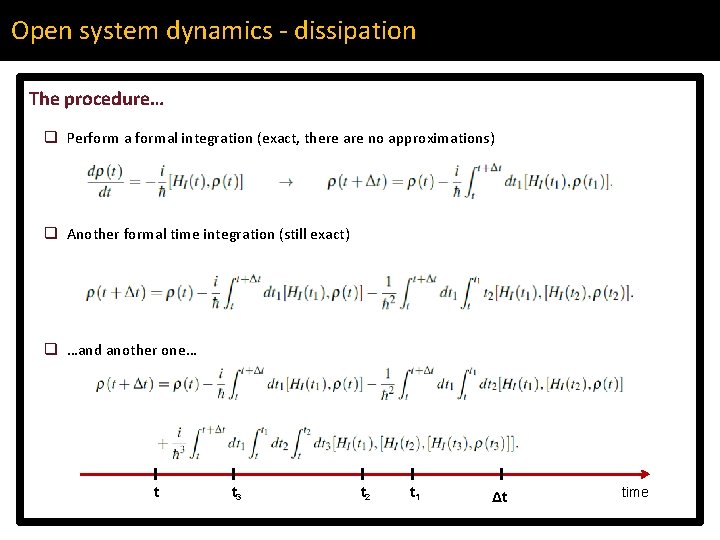
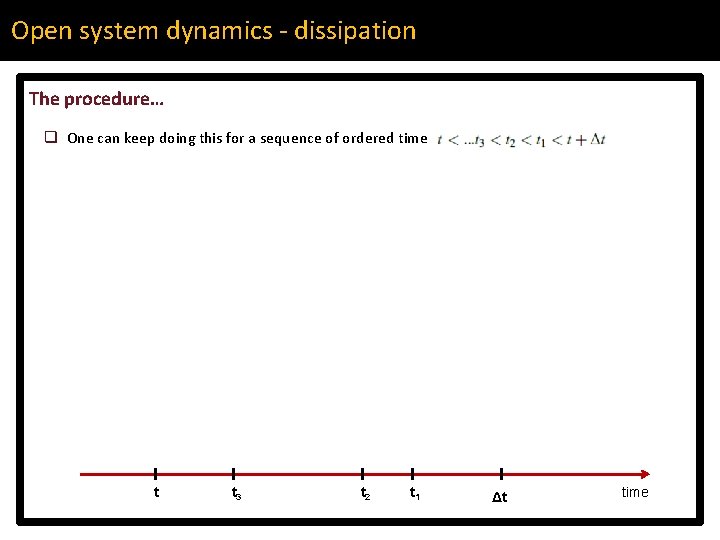
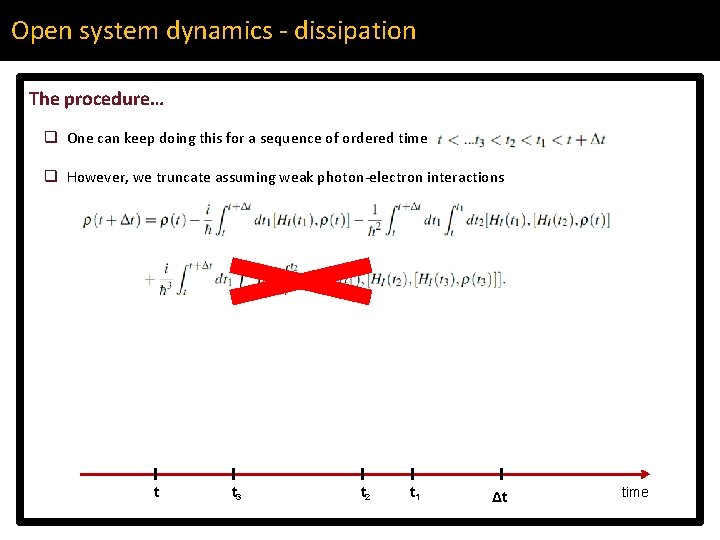
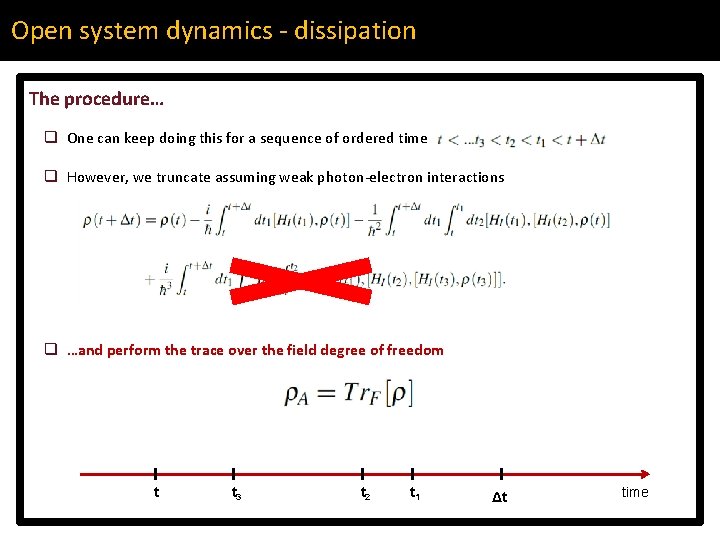
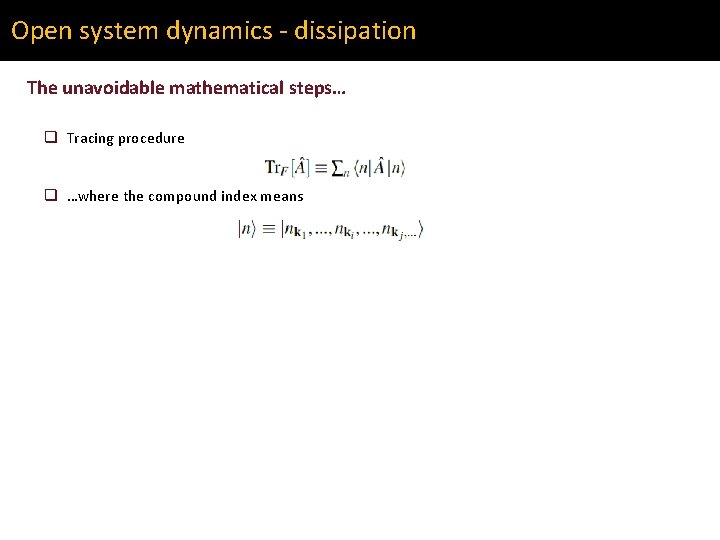
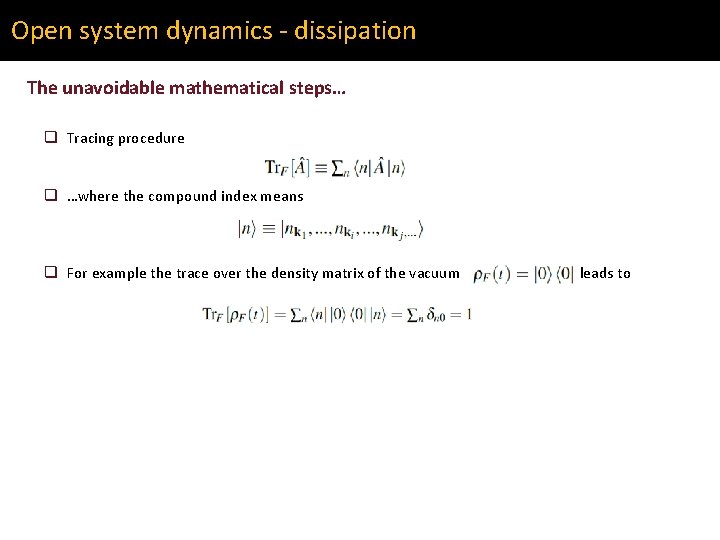
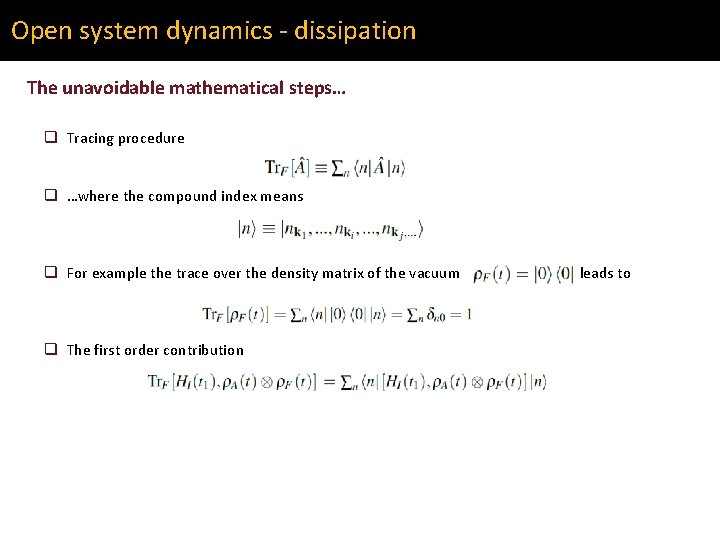
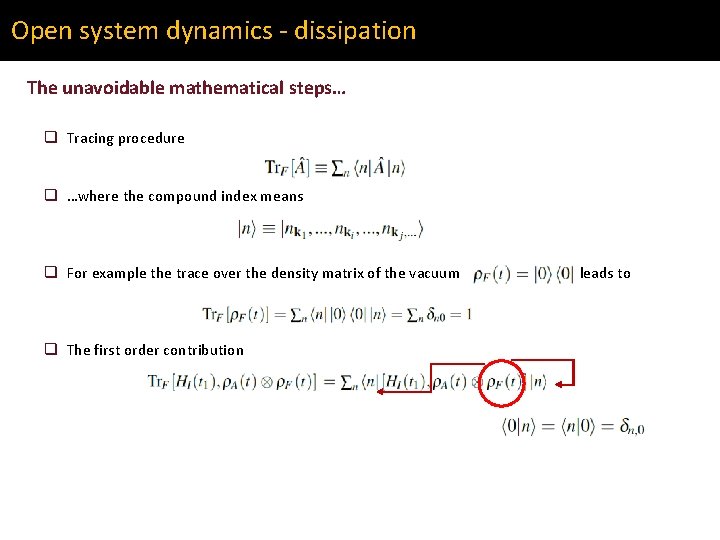
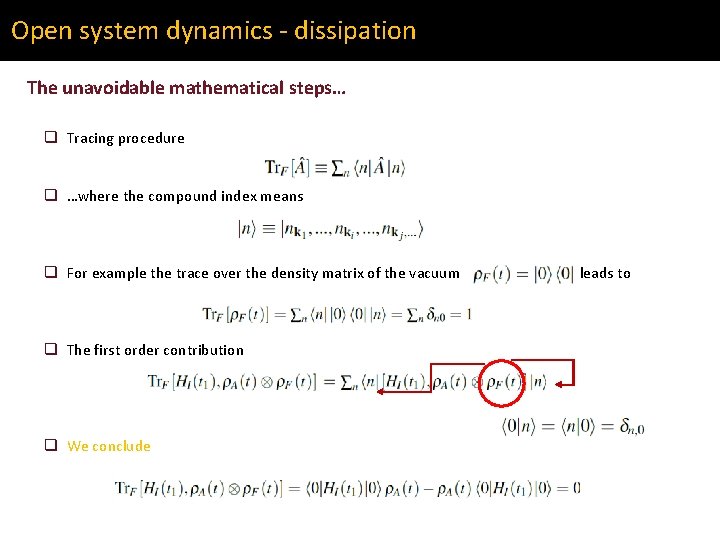
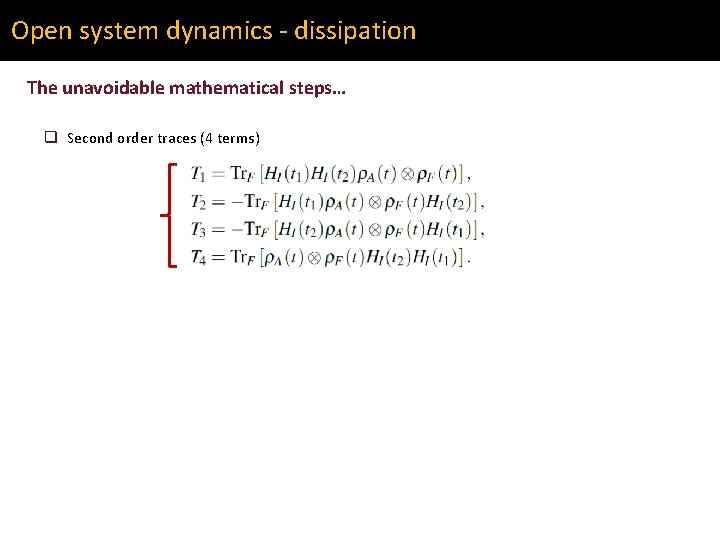
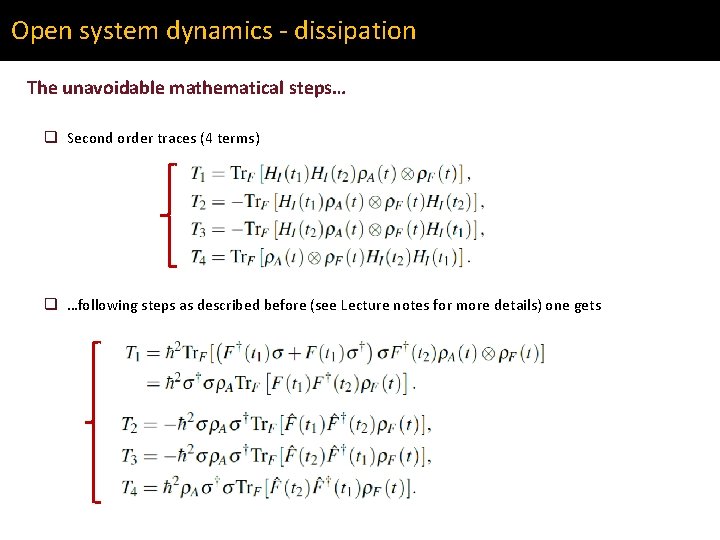
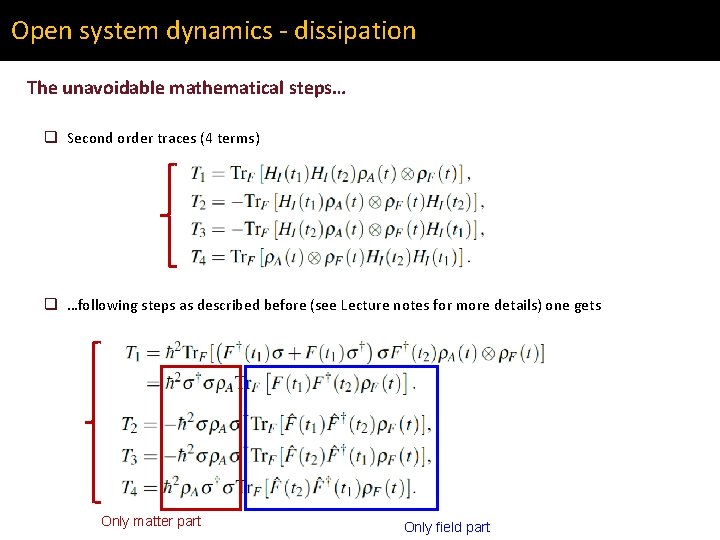
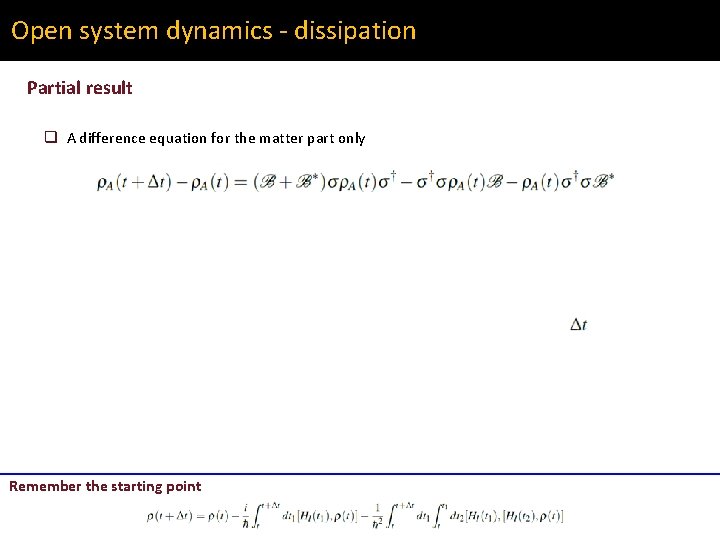
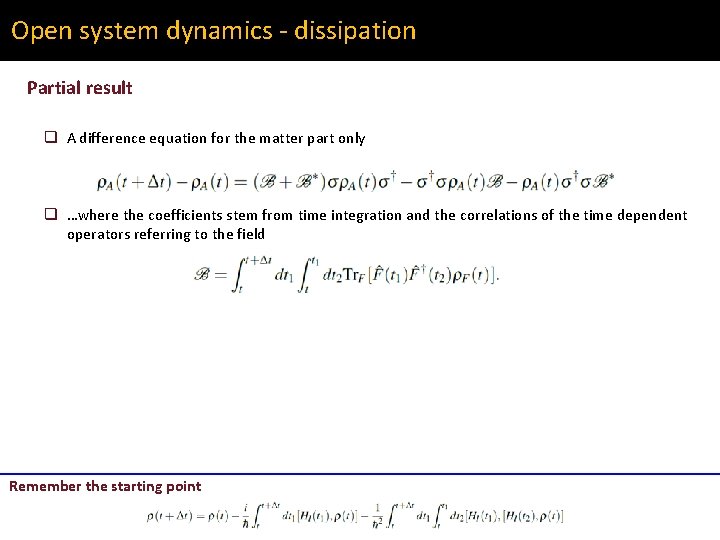
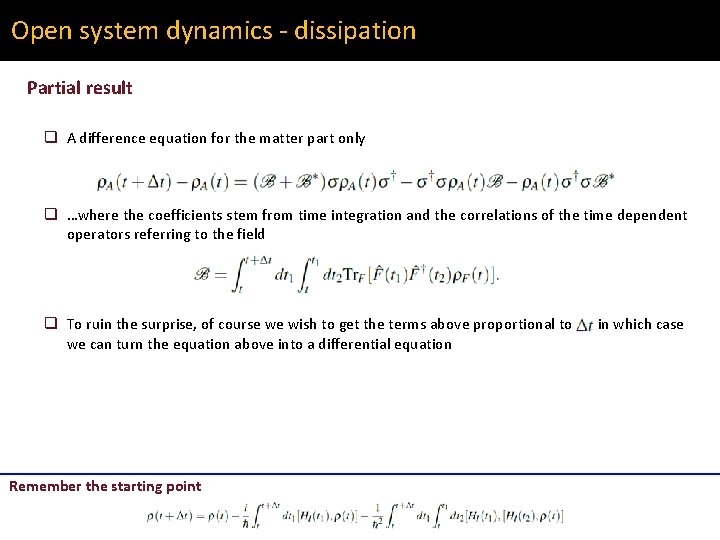
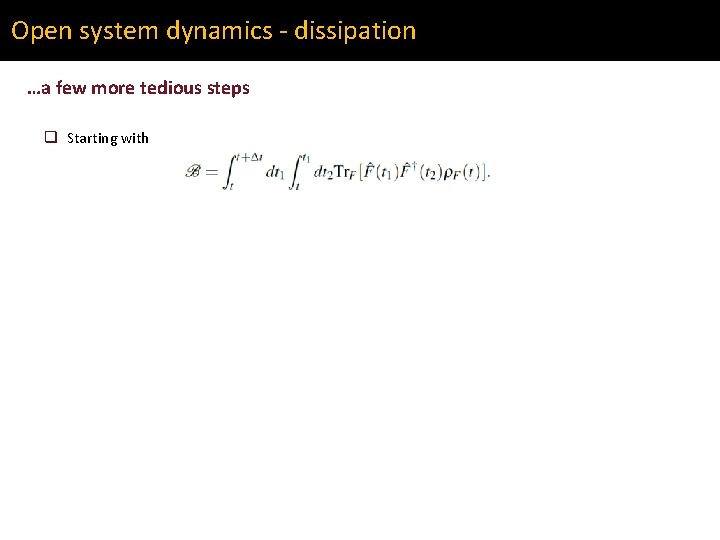
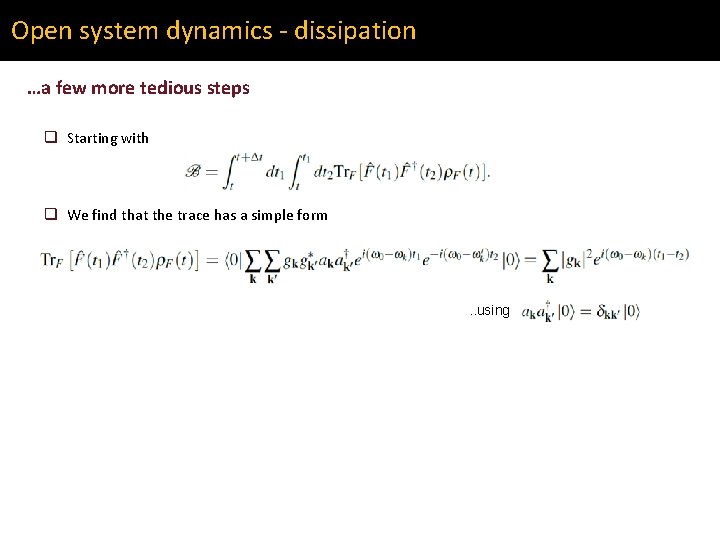
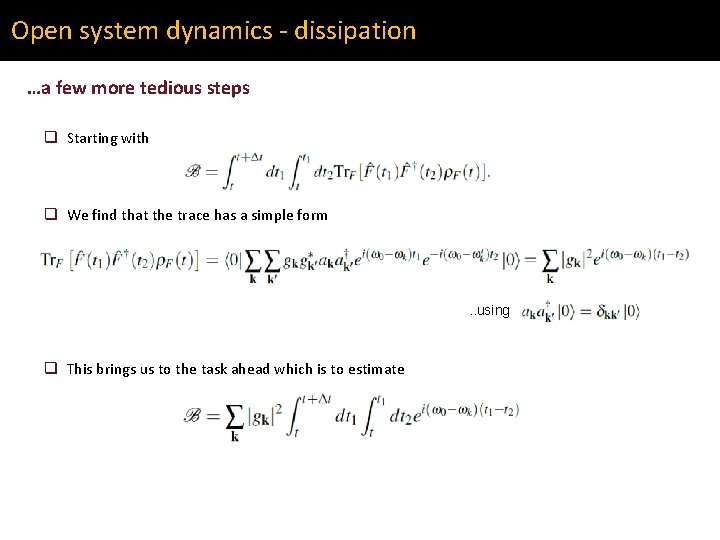
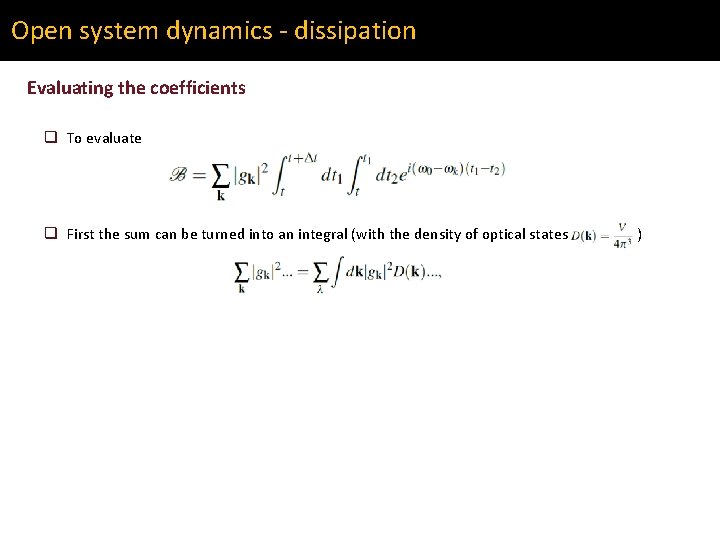
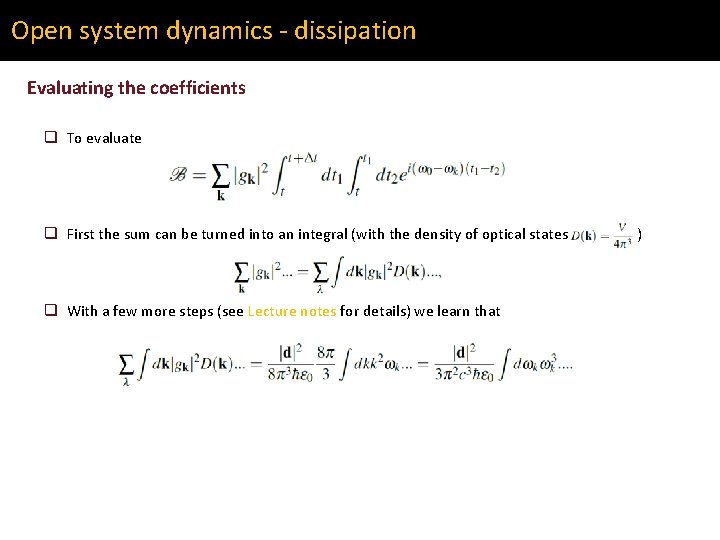
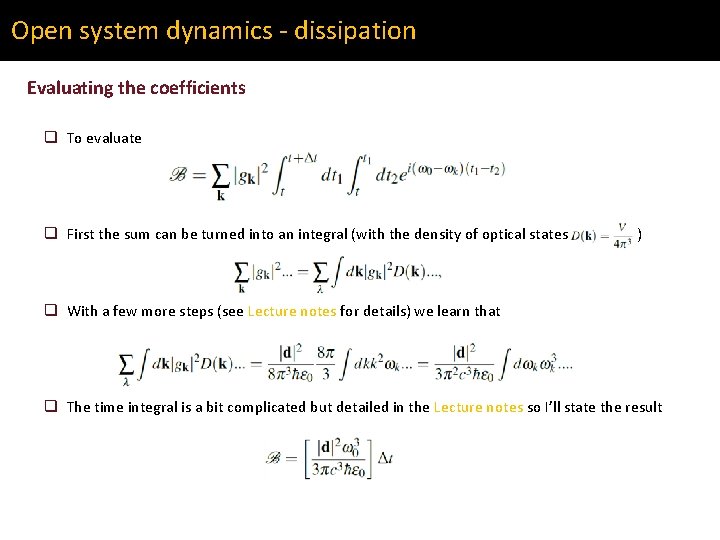
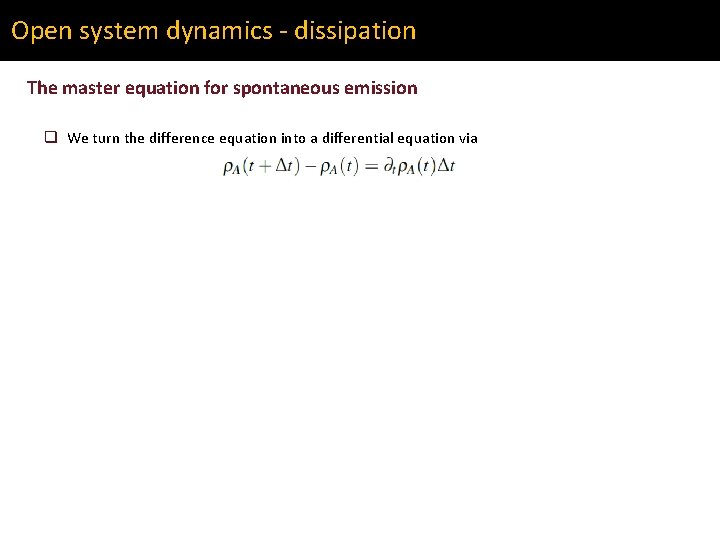
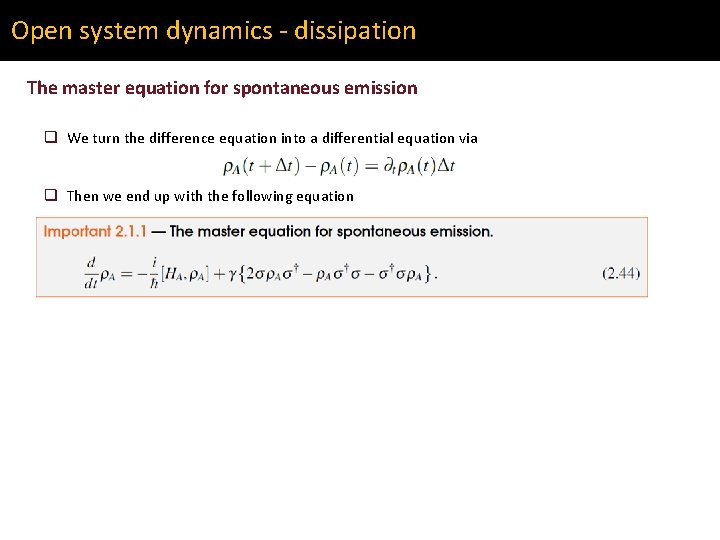
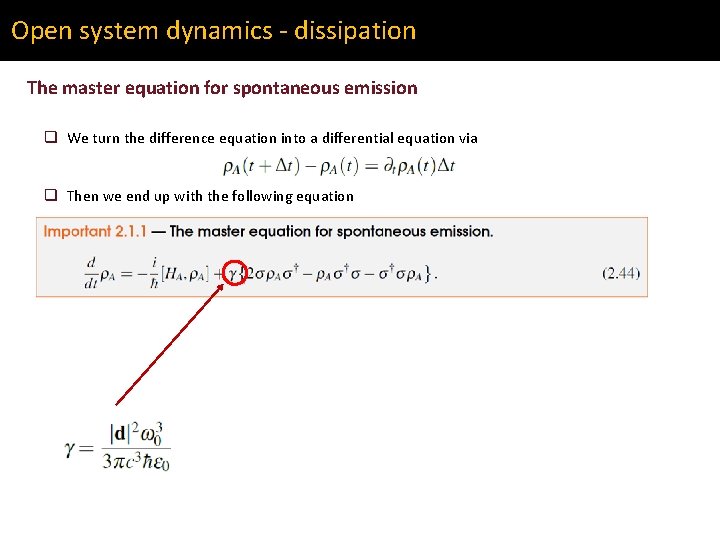
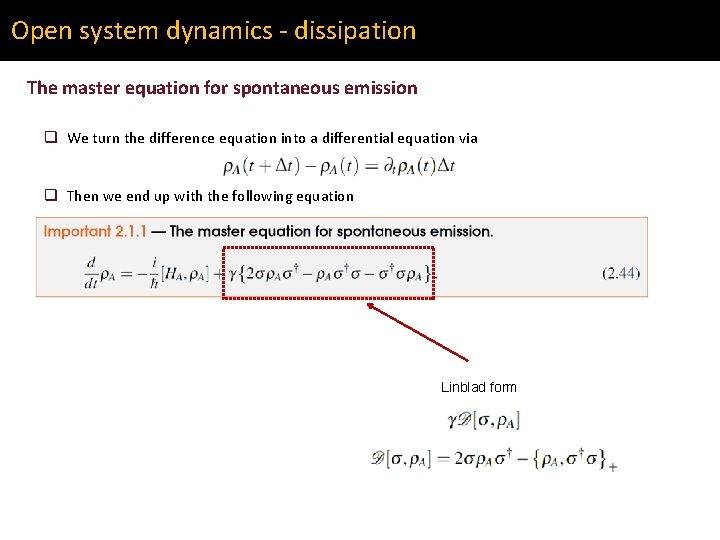
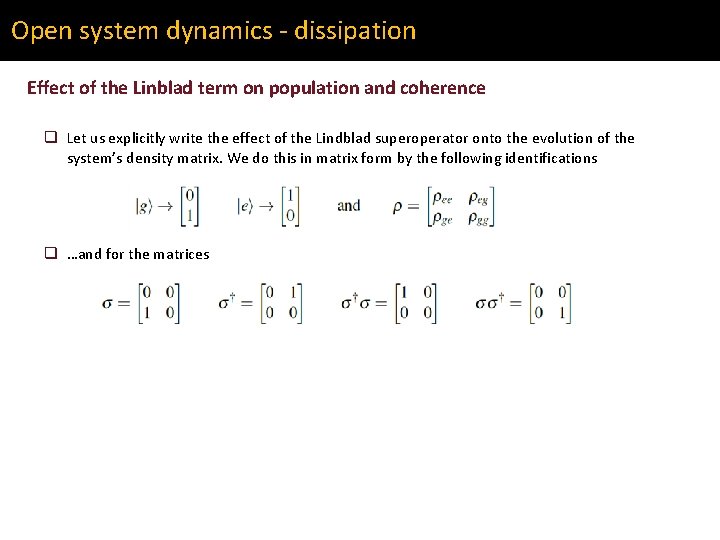
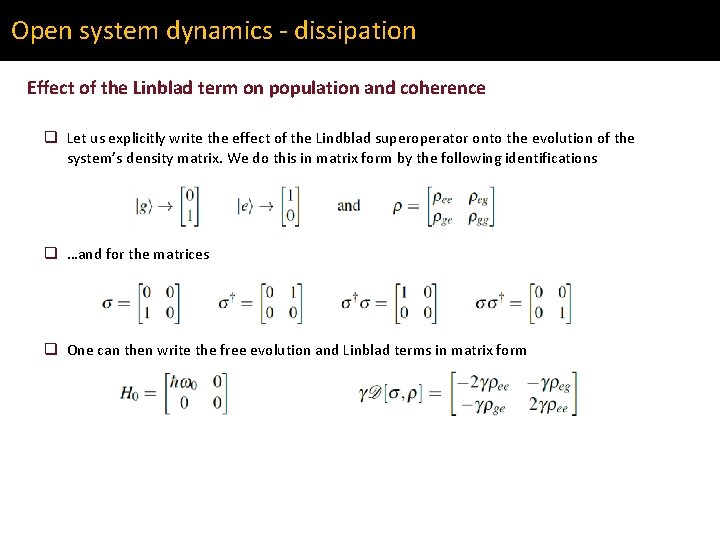
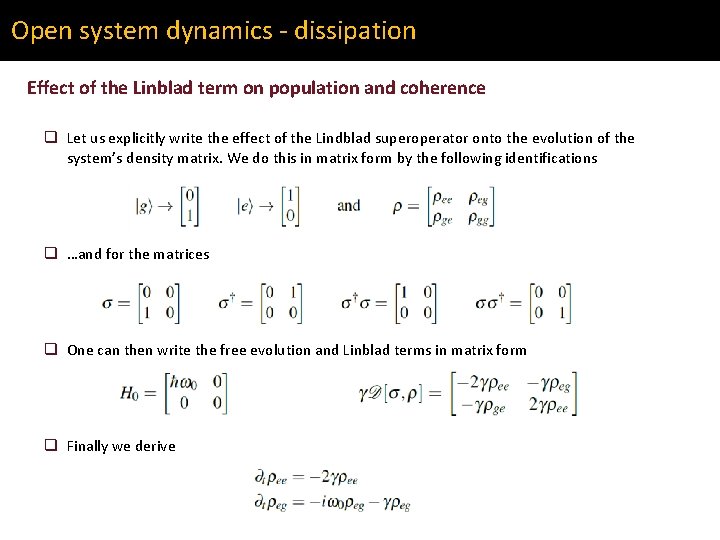
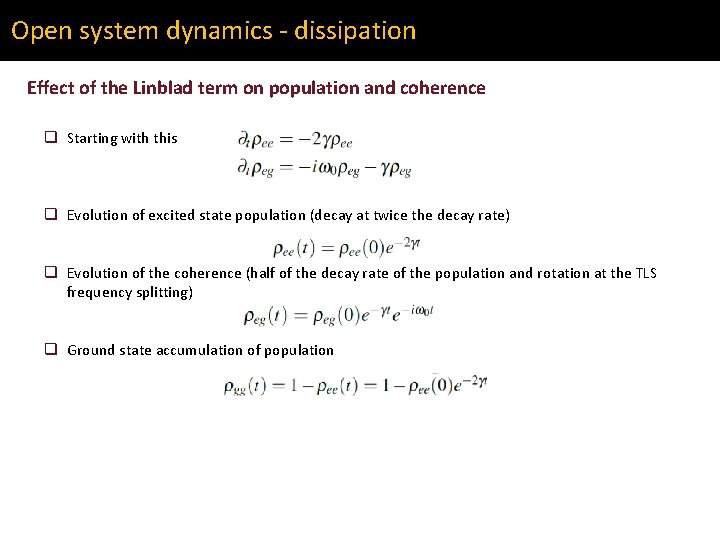
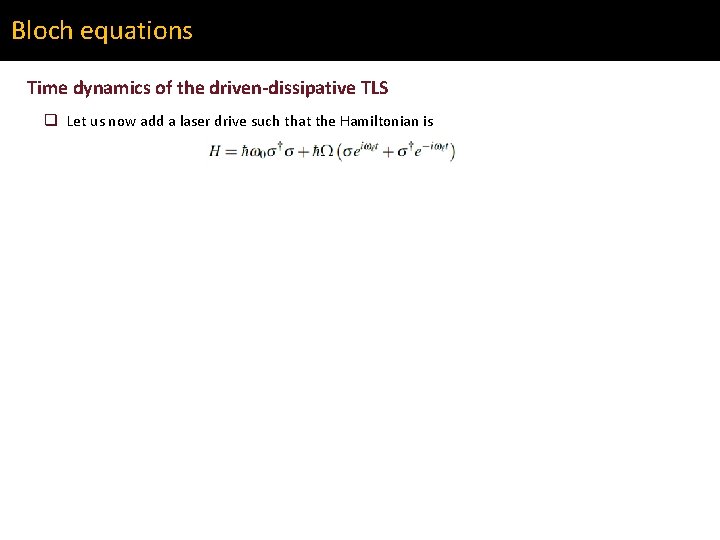
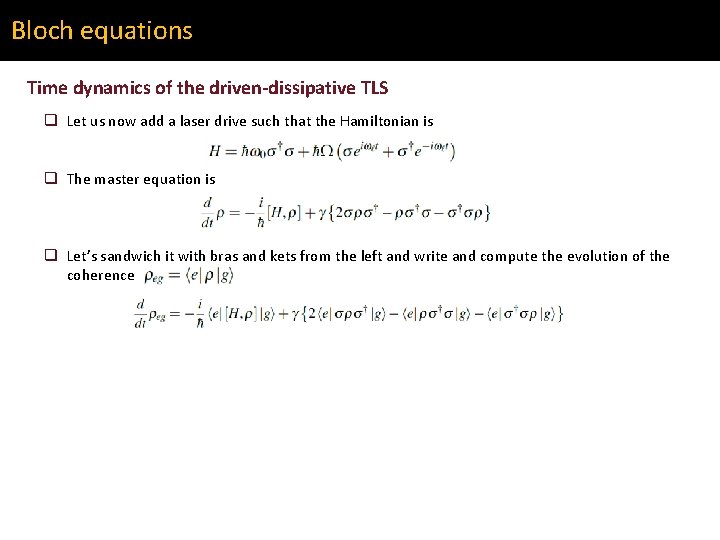
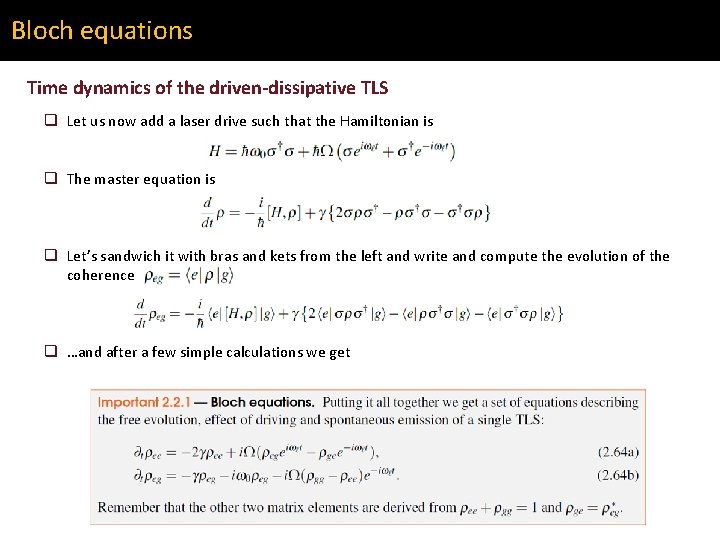
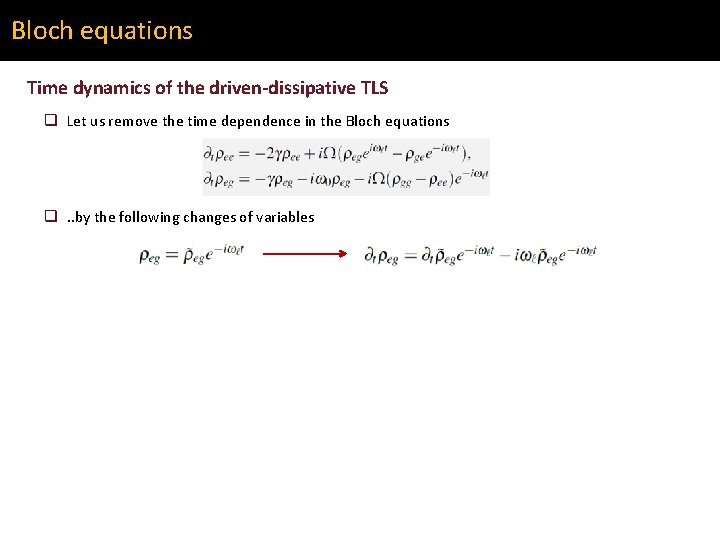
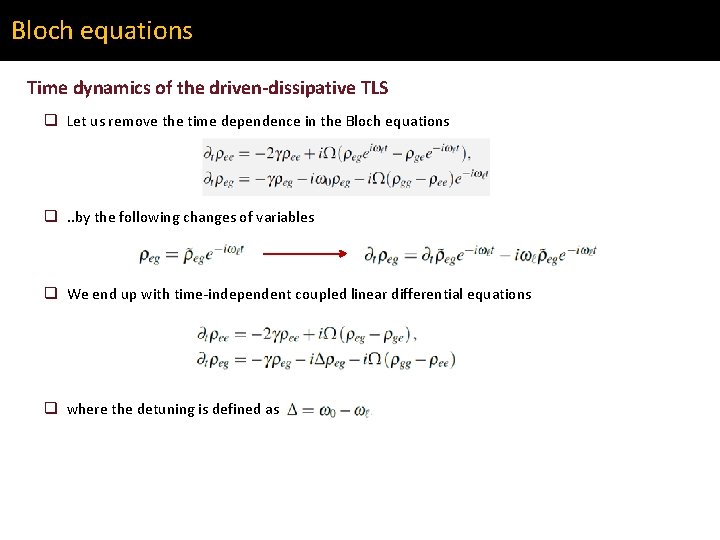
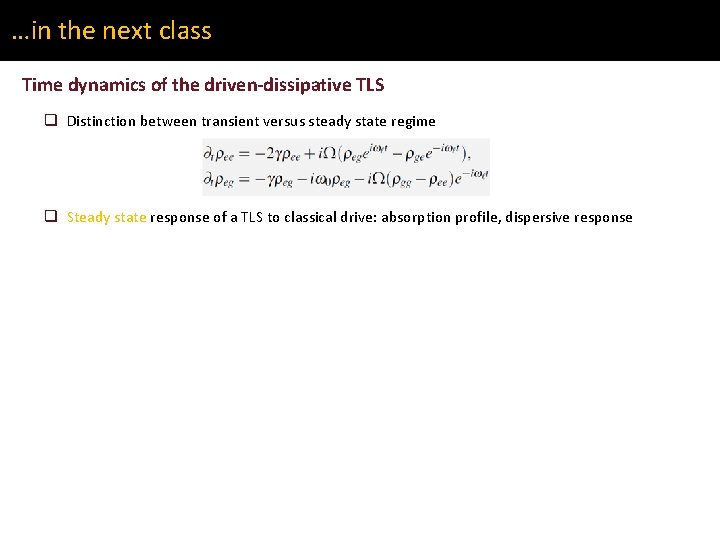
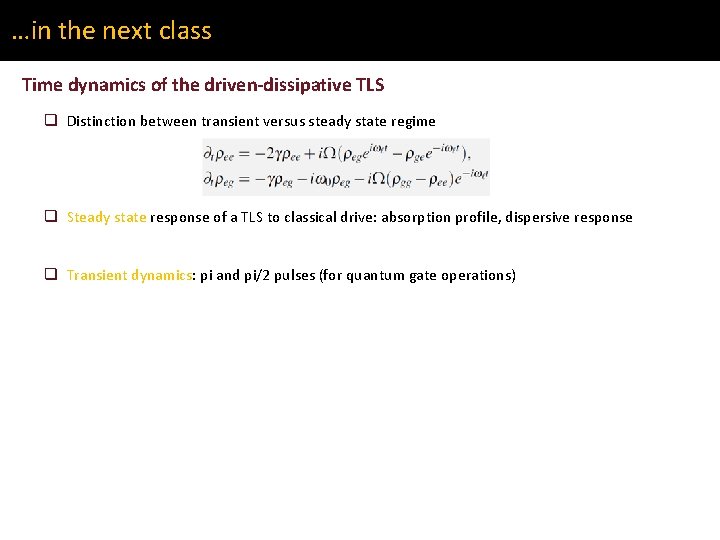
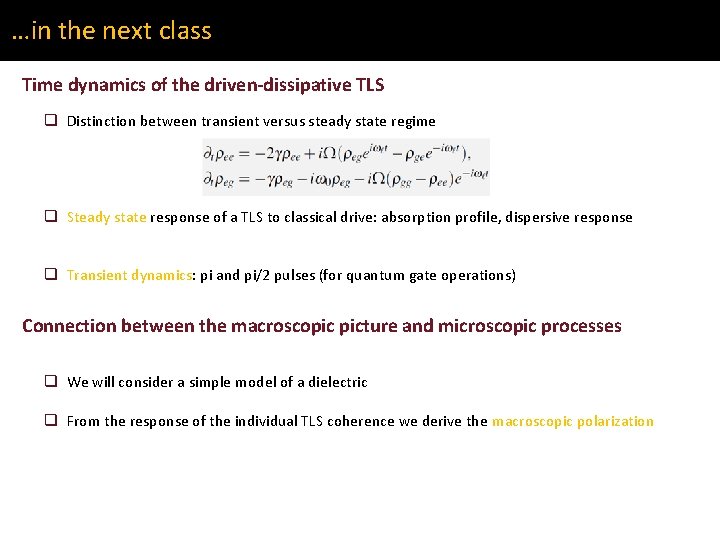
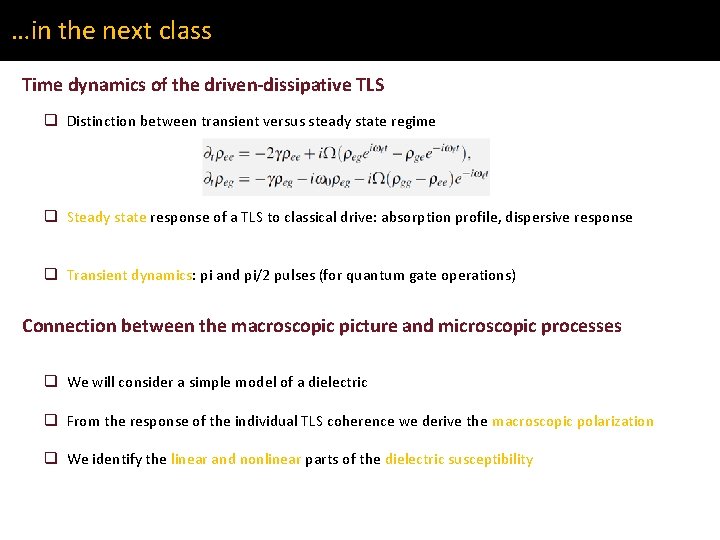
- Slides: 54
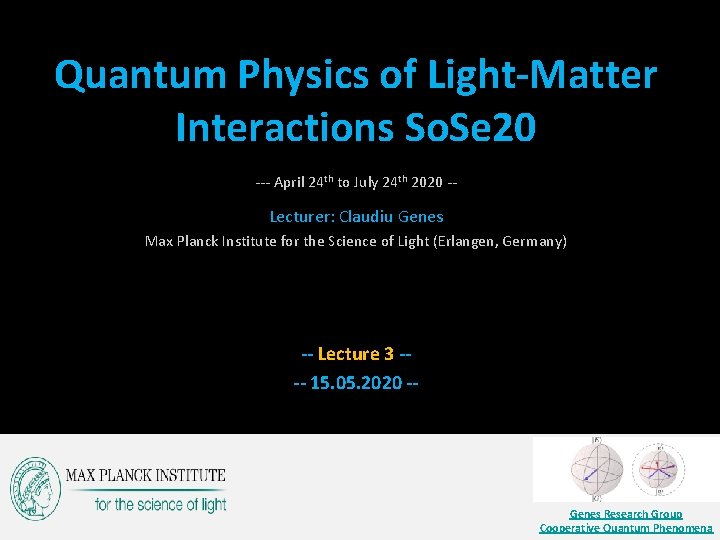
Quantum Physics of Light-Matter Interactions So. Se 20 --- April 24 th to July 24 th 2020 -- Lecturer: Claudiu Genes Max Planck Institute for the Science of Light (Erlangen, Germany) -- Lecture 3 --- 15. 05. 2020 -- Genes Research Group Cooperative Quantum Phenomena
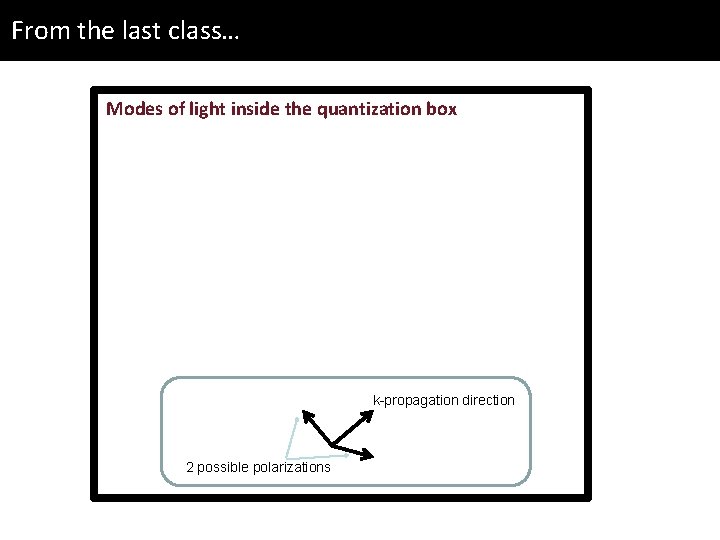
From the last class… Modes of light inside the quantization box k-propagation direction 2 possible polarizations
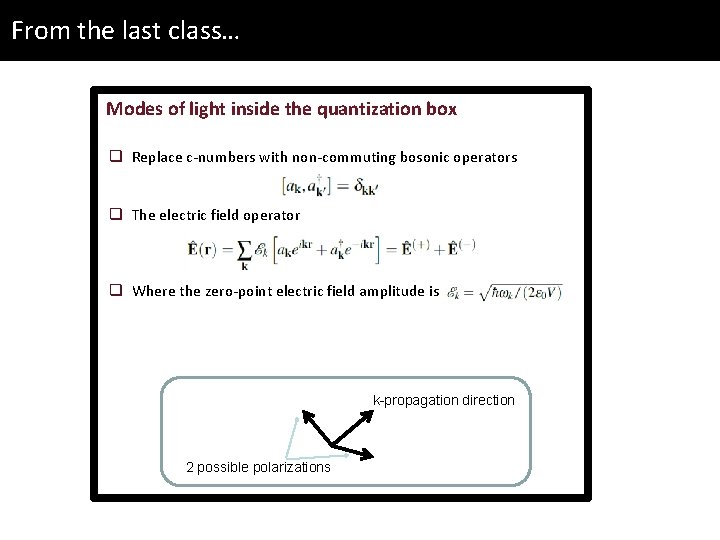
From the last class… Modes of light inside the quantization box q Replace c-numbers with non-commuting bosonic operators q The electric field operator q Where the zero-point electric field amplitude is k-propagation direction 2 possible polarizations
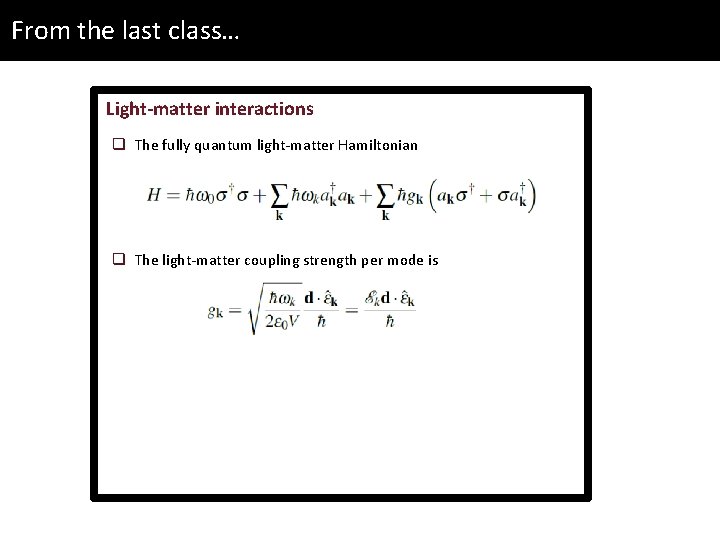
From the last class… Light-matter interactions q The fully quantum light-matter Hamiltonian q The light-matter coupling strength per mode is
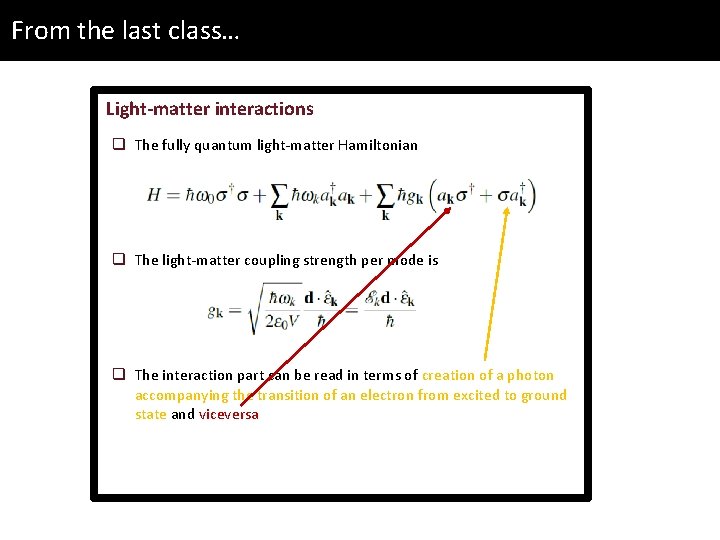
From the last class… Light-matter interactions q The fully quantum light-matter Hamiltonian q The light-matter coupling strength per mode is q The interaction part can be read in terms of creation of a photon accompanying the transition of an electron from excited to ground state and viceversa
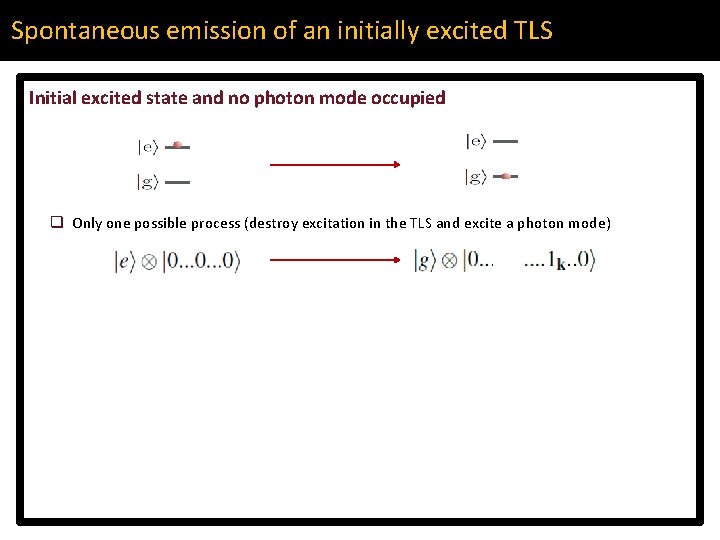
Spontaneous emission of an initially excited TLS Initial excited state and no photon mode occupied q Only one possible process (destroy excitation in the TLS and excite a photon mode)
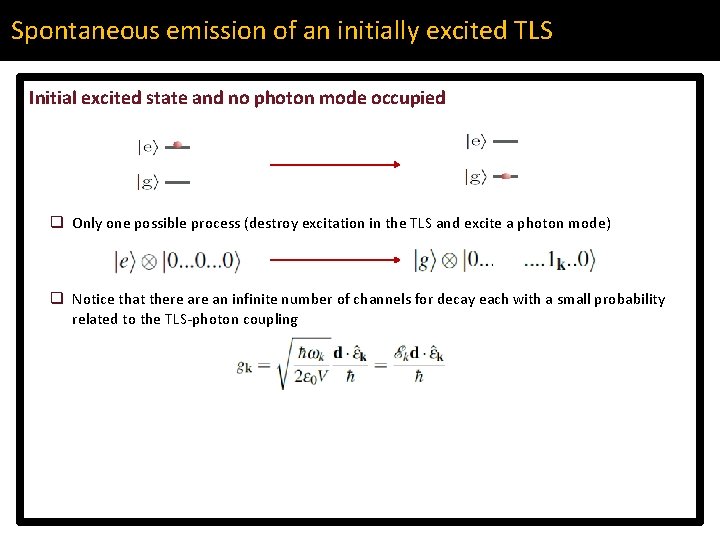
Spontaneous emission of an initially excited TLS Initial excited state and no photon mode occupied q Only one possible process (destroy excitation in the TLS and excite a photon mode) q Notice that there an infinite number of channels for decay each with a small probability related to the TLS-photon coupling
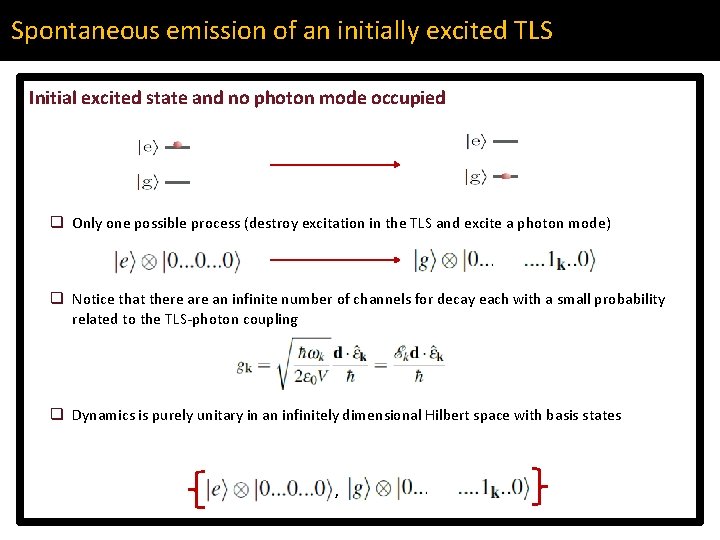
Spontaneous emission of an initially excited TLS Initial excited state and no photon mode occupied q Only one possible process (destroy excitation in the TLS and excite a photon mode) q Notice that there an infinite number of channels for decay each with a small probability related to the TLS-photon coupling q Dynamics is purely unitary in an infinitely dimensional Hilbert space with basis states ,
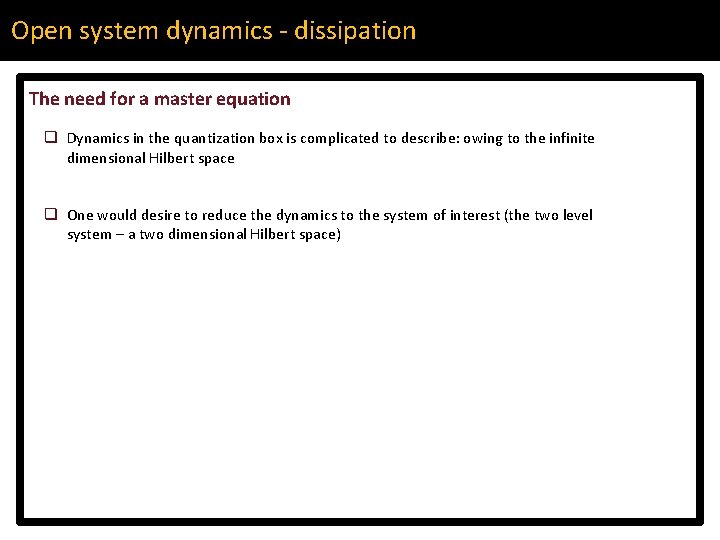
Open system dynamics - dissipation The need for a master equation q Dynamics in the quantization box is complicated to describe: owing to the infinite dimensional Hilbert space q One would desire to reduce the dynamics to the system of interest (the two level system – a two dimensional Hilbert space)
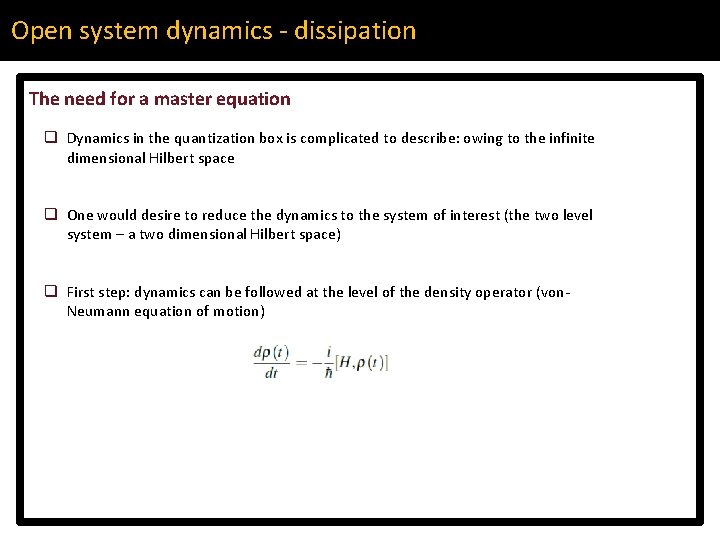
Open system dynamics - dissipation The need for a master equation q Dynamics in the quantization box is complicated to describe: owing to the infinite dimensional Hilbert space q One would desire to reduce the dynamics to the system of interest (the two level system – a two dimensional Hilbert space) q First step: dynamics can be followed at the level of the density operator (von. Neumann equation of motion)
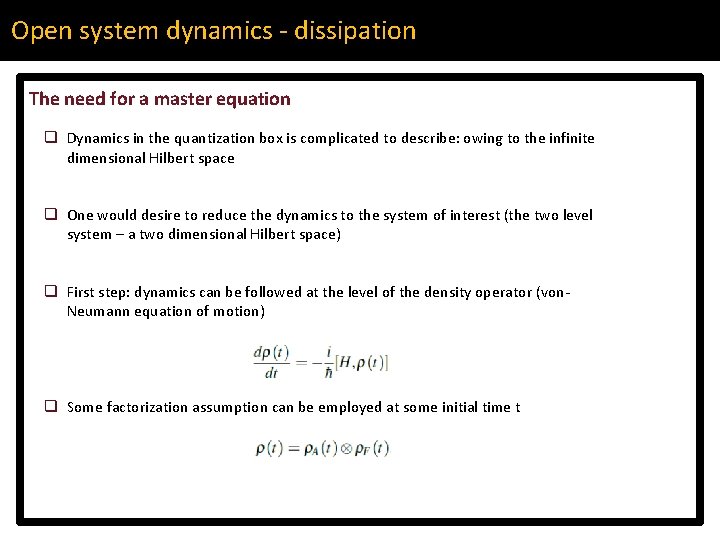
Open system dynamics - dissipation The need for a master equation q Dynamics in the quantization box is complicated to describe: owing to the infinite dimensional Hilbert space q One would desire to reduce the dynamics to the system of interest (the two level system – a two dimensional Hilbert space) q First step: dynamics can be followed at the level of the density operator (von. Neumann equation of motion) q Some factorization assumption can be employed at some initial time t
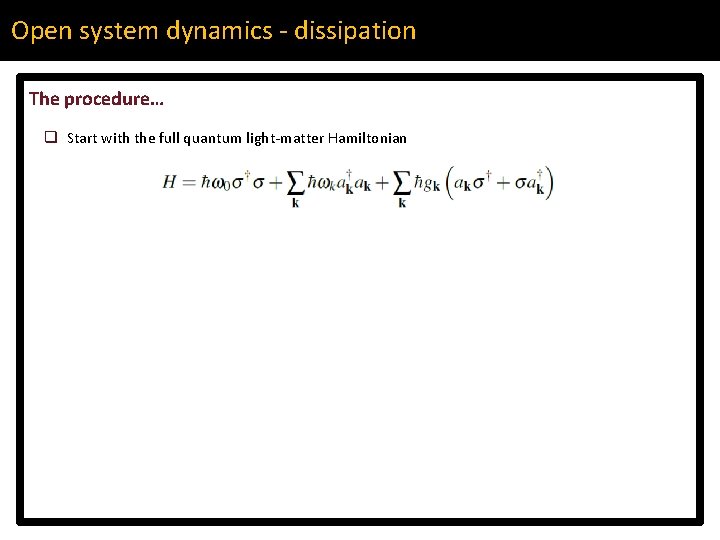
Open system dynamics - dissipation The procedure… q Start with the full quantum light-matter Hamiltonian
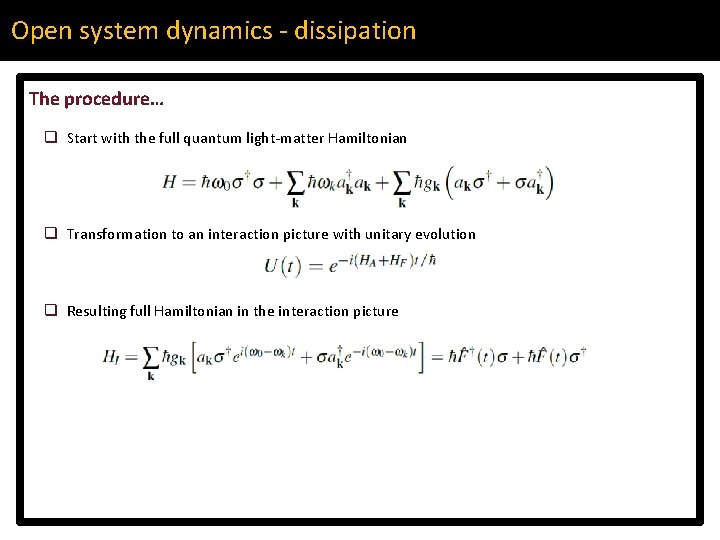
Open system dynamics - dissipation The procedure… q Start with the full quantum light-matter Hamiltonian q Transformation to an interaction picture with unitary evolution q Resulting full Hamiltonian in the interaction picture
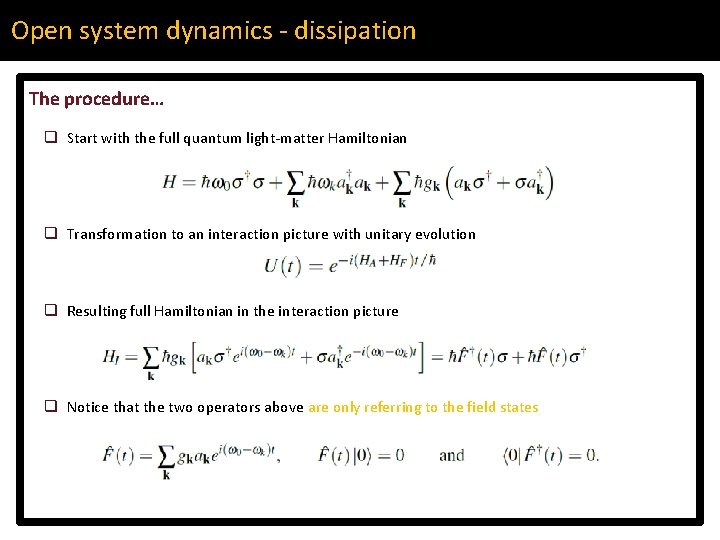
Open system dynamics - dissipation The procedure… q Start with the full quantum light-matter Hamiltonian q Transformation to an interaction picture with unitary evolution q Resulting full Hamiltonian in the interaction picture q Notice that the two operators above are only referring to the field states
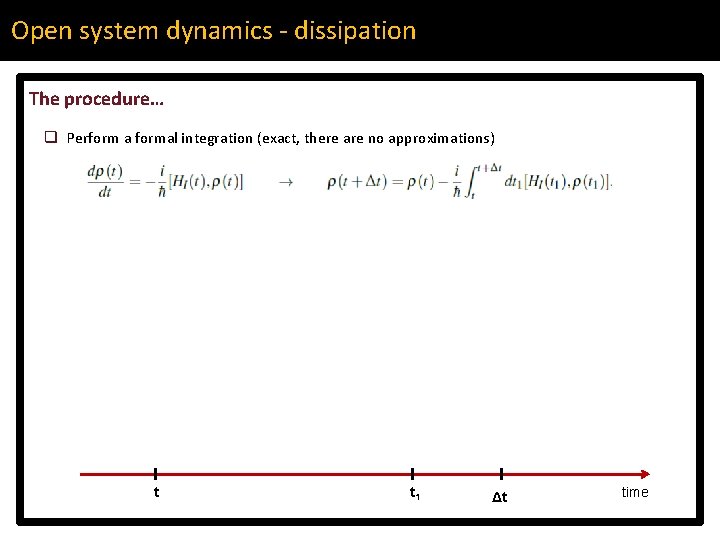
Open system dynamics - dissipation The procedure… q Perform a formal integration (exact, there are no approximations) t t 1 Δt time
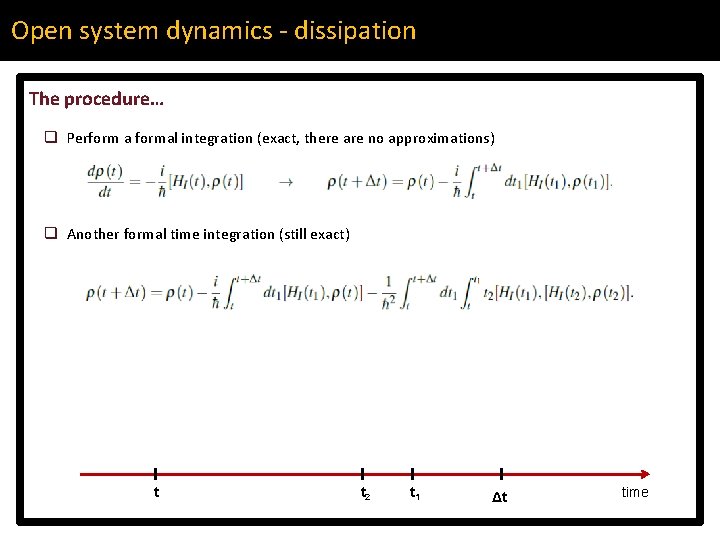
Open system dynamics - dissipation The procedure… q Perform a formal integration (exact, there are no approximations) q Another formal time integration (still exact) t t 2 t 1 Δt time
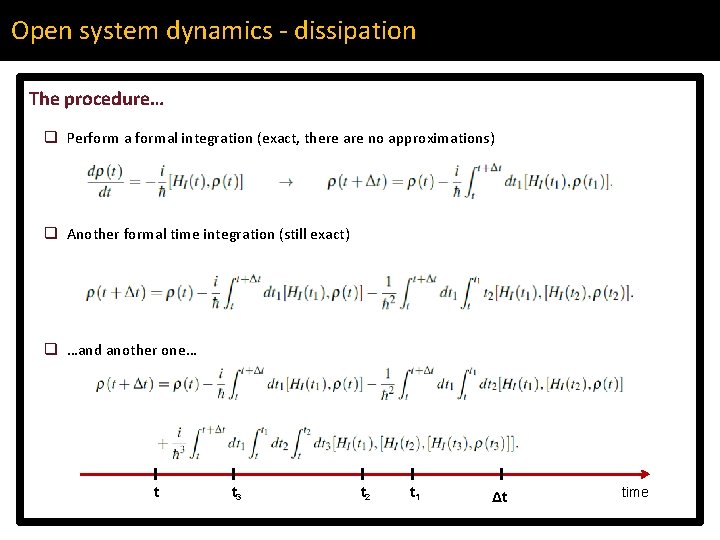
Open system dynamics - dissipation The procedure… q Perform a formal integration (exact, there are no approximations) q Another formal time integration (still exact) q …and another one… t t 3 t 2 t 1 Δt time
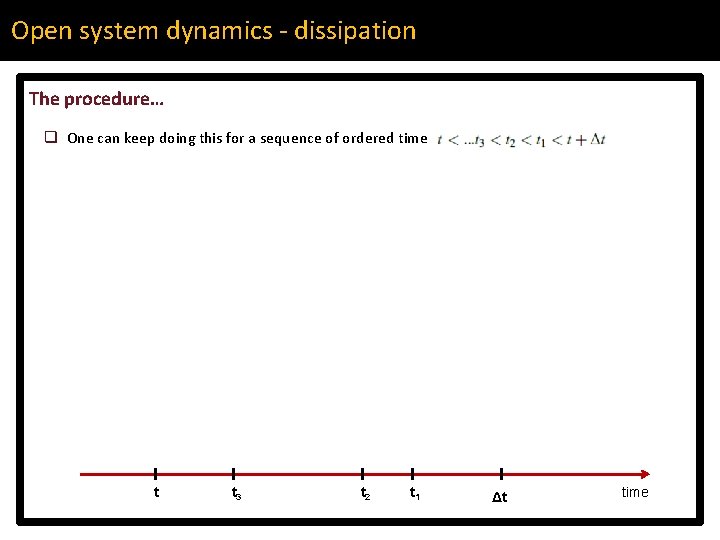
Open system dynamics - dissipation The procedure… q One can keep doing this for a sequence of ordered time t t 3 t 2 t 1 Δt time
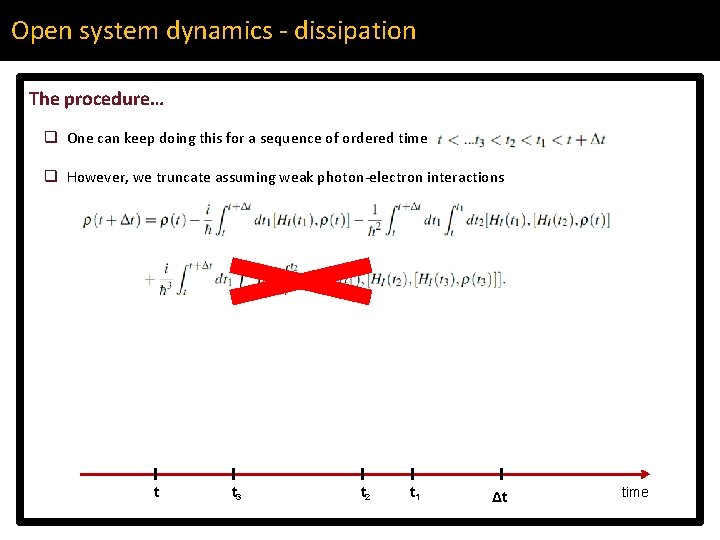
Open system dynamics - dissipation The procedure… q One can keep doing this for a sequence of ordered time q However, we truncate assuming weak photon-electron interactions t t 3 t 2 t 1 Δt time
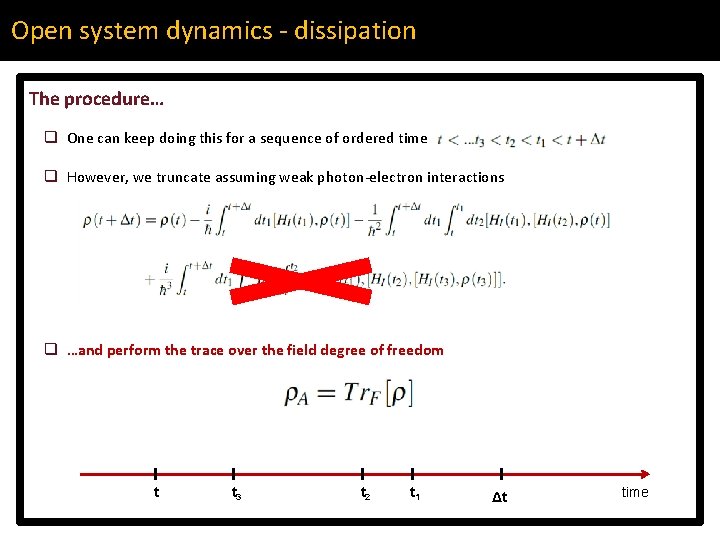
Open system dynamics - dissipation The procedure… q One can keep doing this for a sequence of ordered time q However, we truncate assuming weak photon-electron interactions q …and perform the trace over the field degree of freedom t t 3 t 2 t 1 Δt time
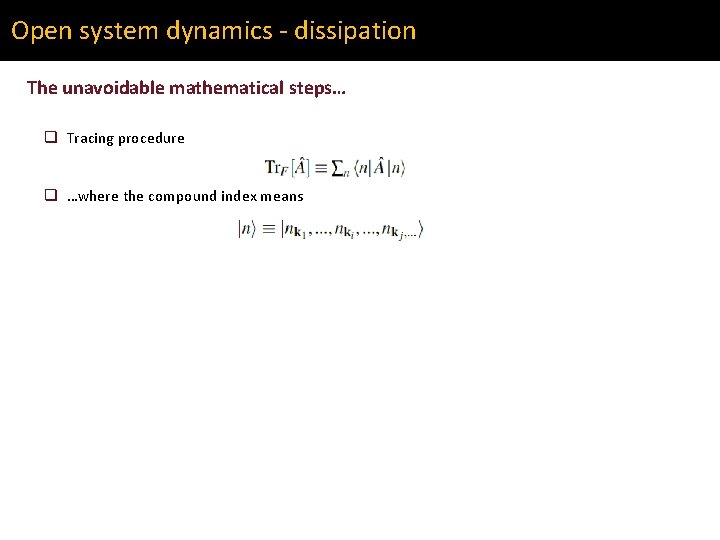
Open system dynamics - dissipation The unavoidable mathematical steps… q Tracing procedure q …where the compound index means
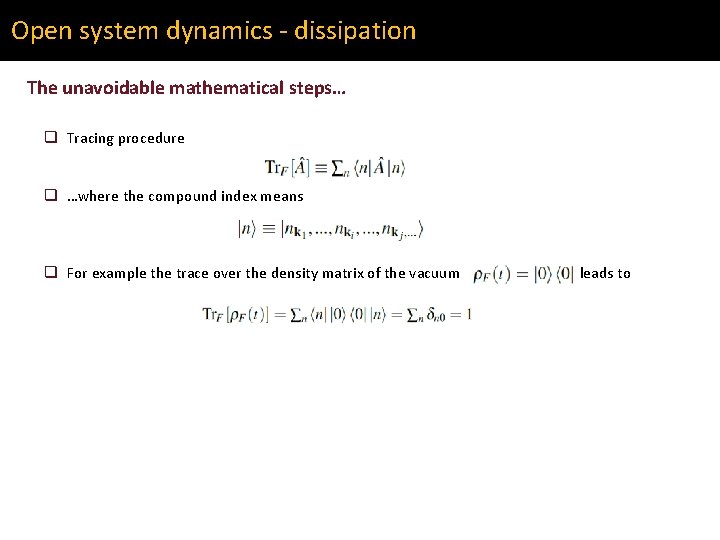
Open system dynamics - dissipation The unavoidable mathematical steps… q Tracing procedure q …where the compound index means q For example the trace over the density matrix of the vacuum leads to
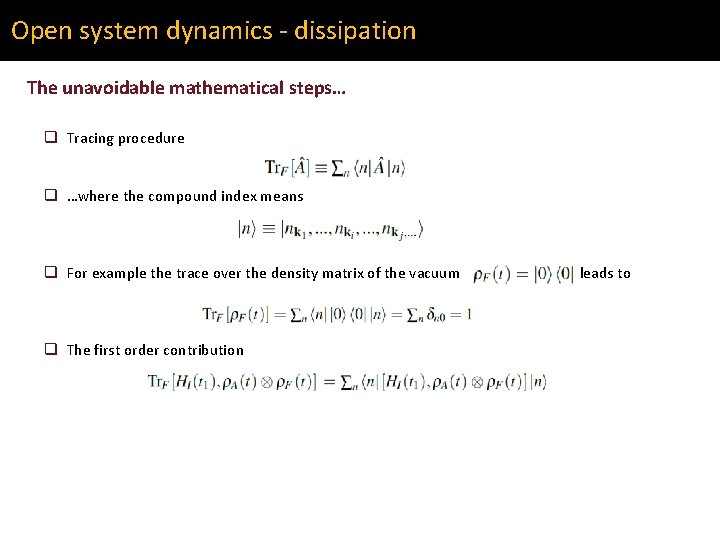
Open system dynamics - dissipation The unavoidable mathematical steps… q Tracing procedure q …where the compound index means q For example the trace over the density matrix of the vacuum q The first order contribution leads to
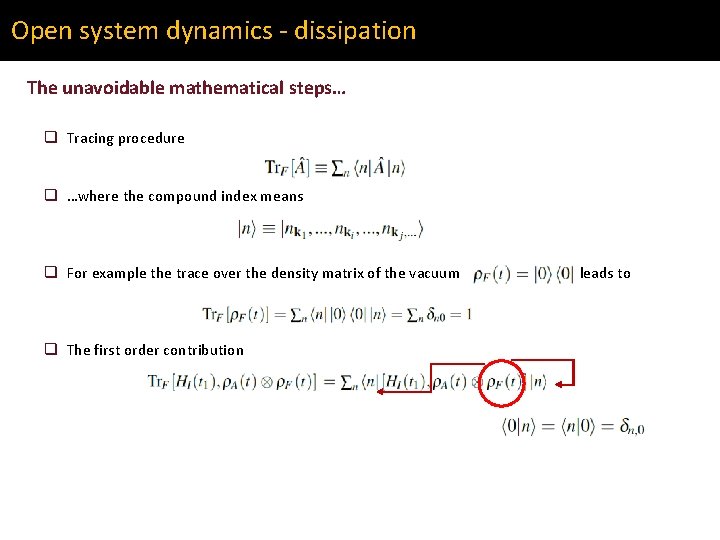
Open system dynamics - dissipation The unavoidable mathematical steps… q Tracing procedure q …where the compound index means q For example the trace over the density matrix of the vacuum q The first order contribution leads to
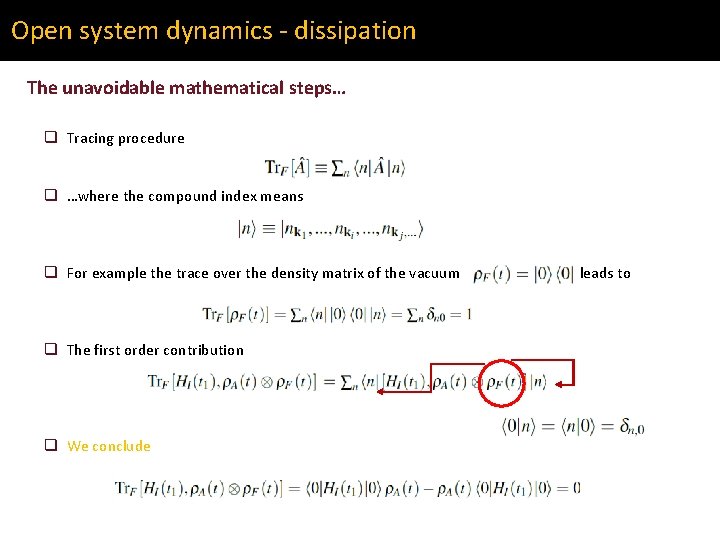
Open system dynamics - dissipation The unavoidable mathematical steps… q Tracing procedure q …where the compound index means q For example the trace over the density matrix of the vacuum q The first order contribution q We conclude leads to
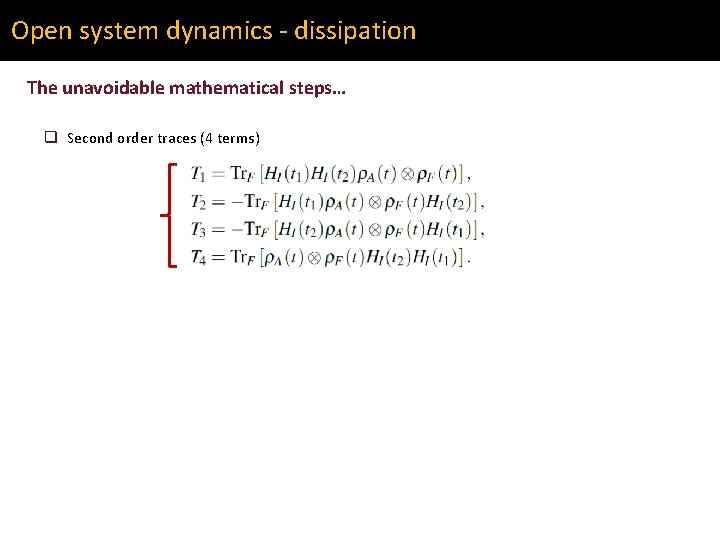
Open system dynamics - dissipation The unavoidable mathematical steps… q Second order traces (4 terms)
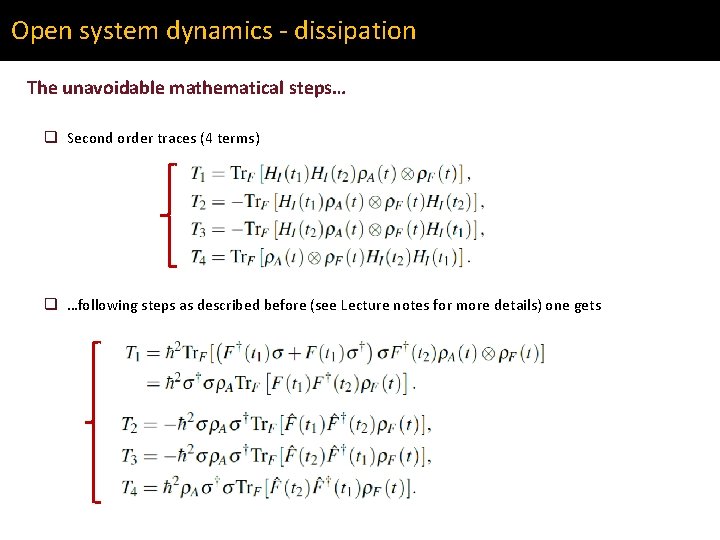
Open system dynamics - dissipation The unavoidable mathematical steps… q Second order traces (4 terms) q …following steps as described before (see Lecture notes for more details) one gets
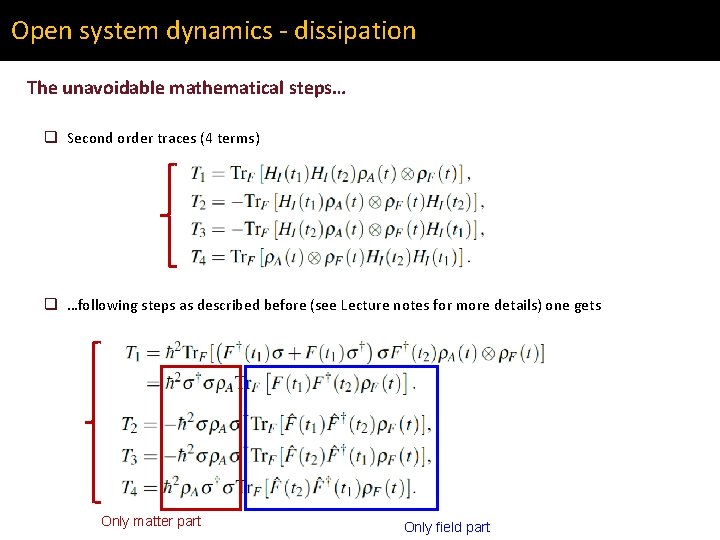
Open system dynamics - dissipation The unavoidable mathematical steps… q Second order traces (4 terms) q …following steps as described before (see Lecture notes for more details) one gets Only matter part Only field part
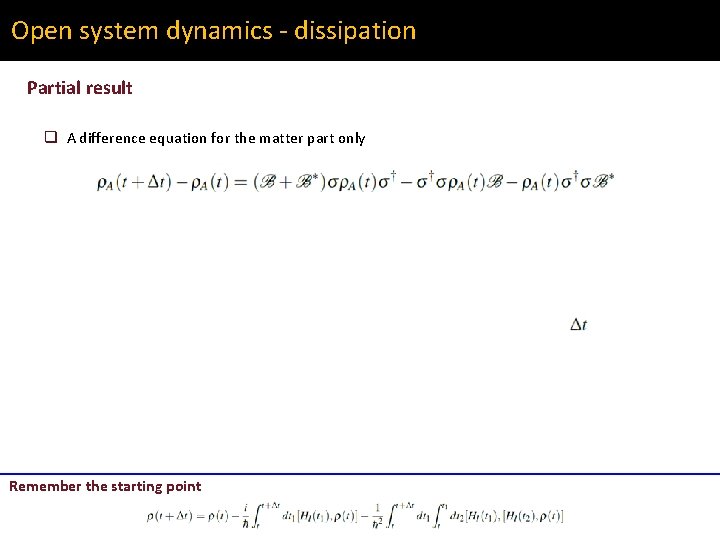
Open system dynamics - dissipation Partial result q A difference equation for the matter part only Remember the starting point
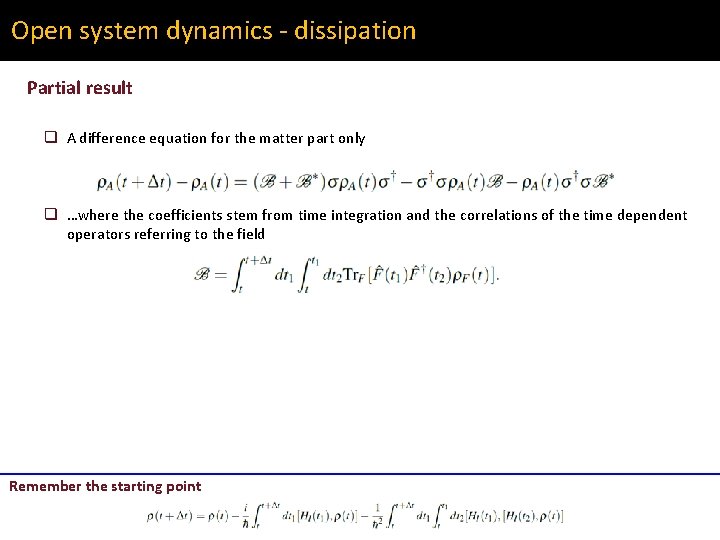
Open system dynamics - dissipation Partial result q A difference equation for the matter part only q …where the coefficients stem from time integration and the correlations of the time dependent operators referring to the field Remember the starting point
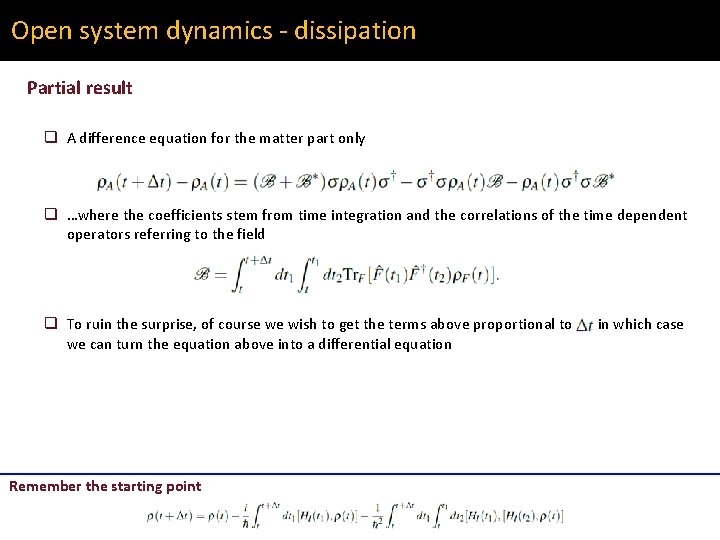
Open system dynamics - dissipation Partial result q A difference equation for the matter part only q …where the coefficients stem from time integration and the correlations of the time dependent operators referring to the field q To ruin the surprise, of course we wish to get the terms above proportional to we can turn the equation above into a differential equation Remember the starting point in which case
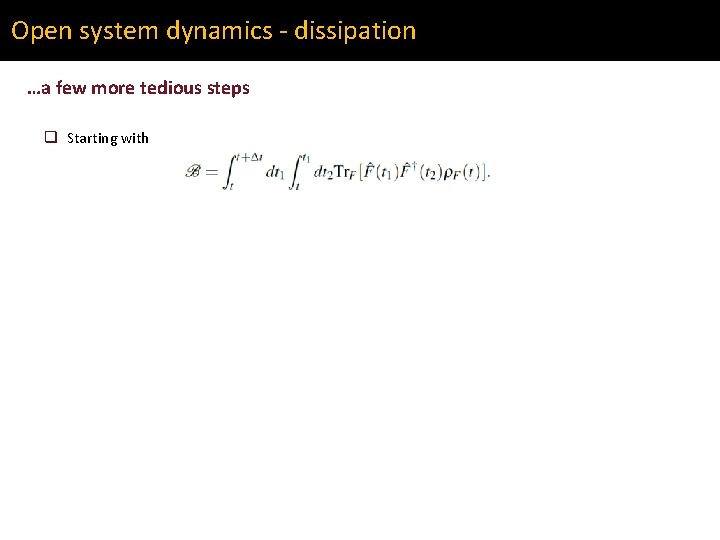
Open system dynamics - dissipation …a few more tedious steps q Starting with
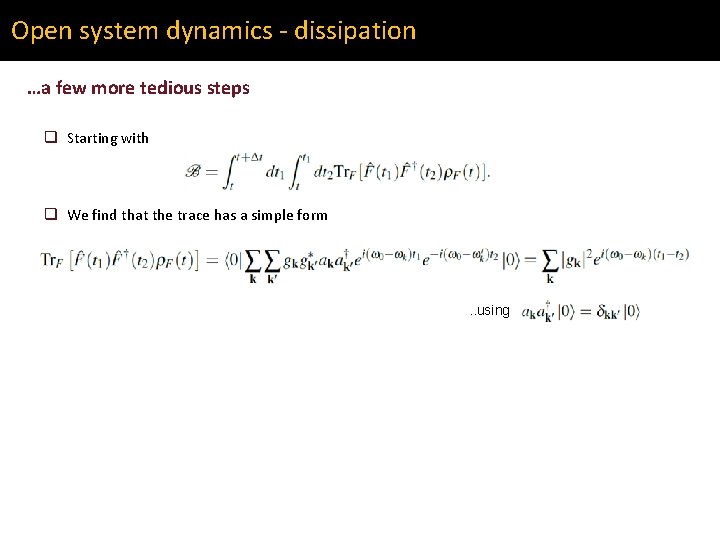
Open system dynamics - dissipation …a few more tedious steps q Starting with q We find that the trace has a simple form . . using
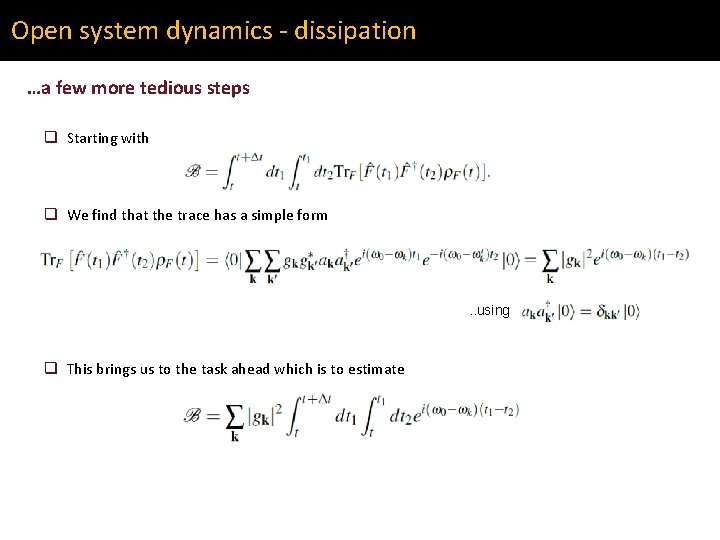
Open system dynamics - dissipation …a few more tedious steps q Starting with q We find that the trace has a simple form . . using q This brings us to the task ahead which is to estimate
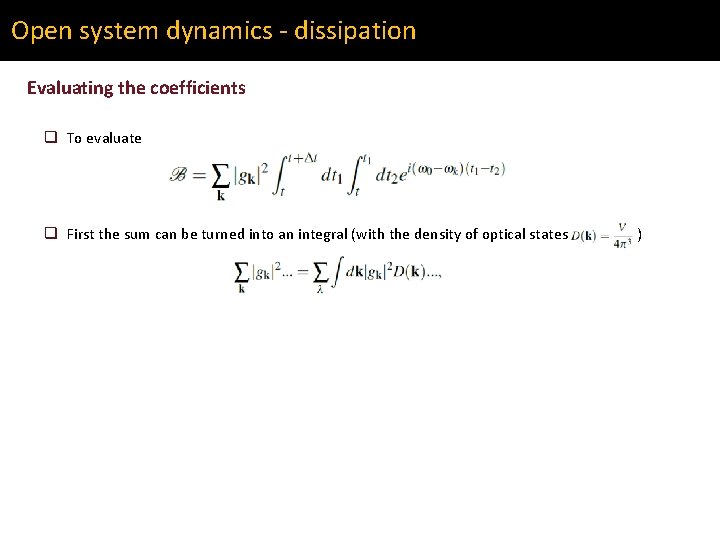
Open system dynamics - dissipation Evaluating the coefficients q To evaluate q First the sum can be turned into an integral (with the density of optical states )
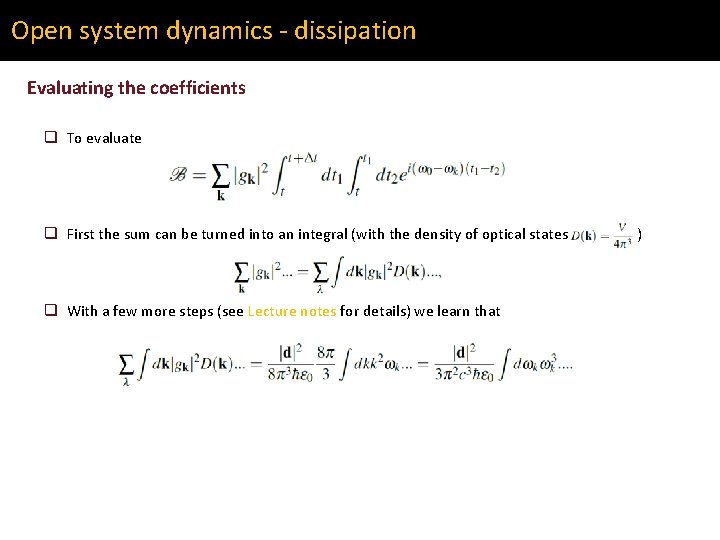
Open system dynamics - dissipation Evaluating the coefficients q To evaluate q First the sum can be turned into an integral (with the density of optical states q With a few more steps (see Lecture notes for details) we learn that )
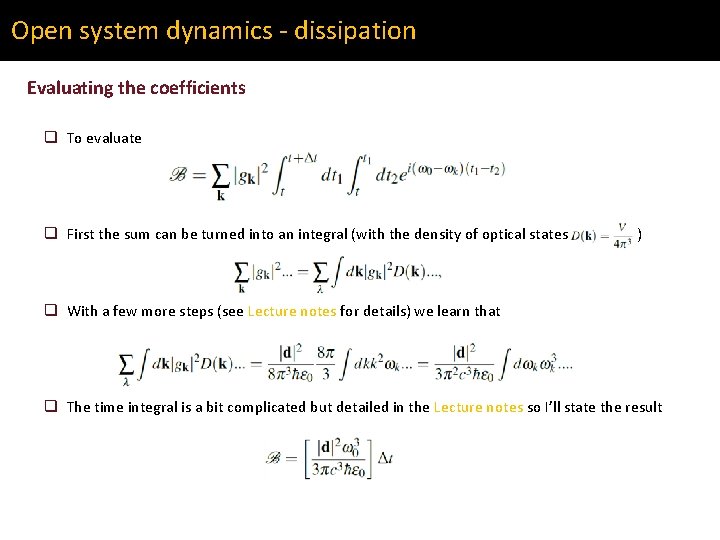
Open system dynamics - dissipation Evaluating the coefficients q To evaluate q First the sum can be turned into an integral (with the density of optical states ) q With a few more steps (see Lecture notes for details) we learn that q The time integral is a bit complicated but detailed in the Lecture notes so I’ll state the result
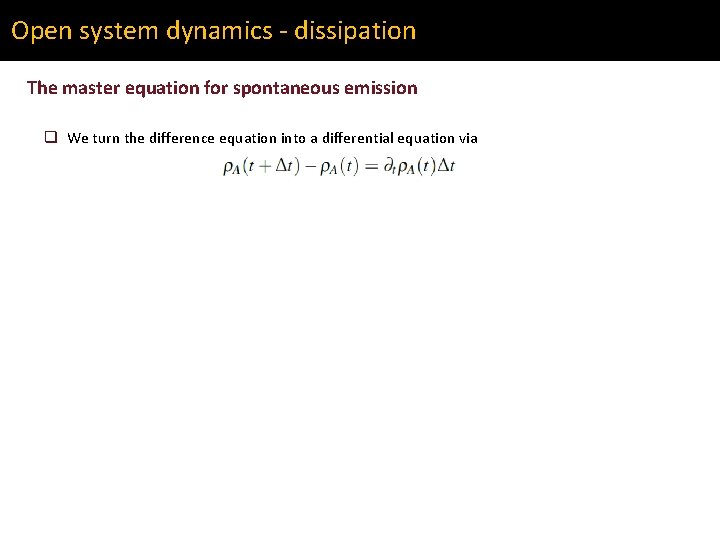
Open system dynamics - dissipation The master equation for spontaneous emission q We turn the difference equation into a differential equation via
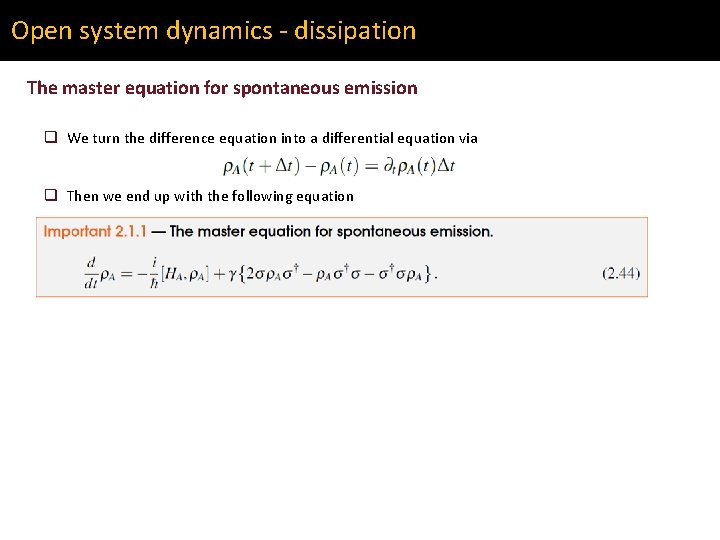
Open system dynamics - dissipation The master equation for spontaneous emission q We turn the difference equation into a differential equation via q Then we end up with the following equation
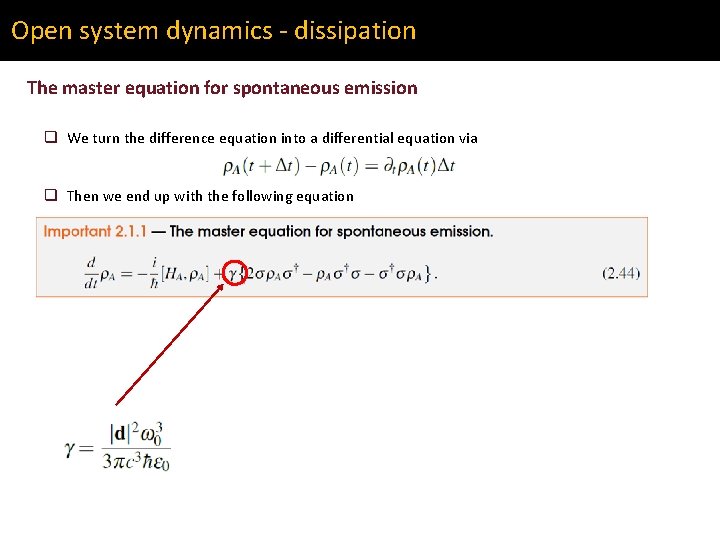
Open system dynamics - dissipation The master equation for spontaneous emission q We turn the difference equation into a differential equation via q Then we end up with the following equation
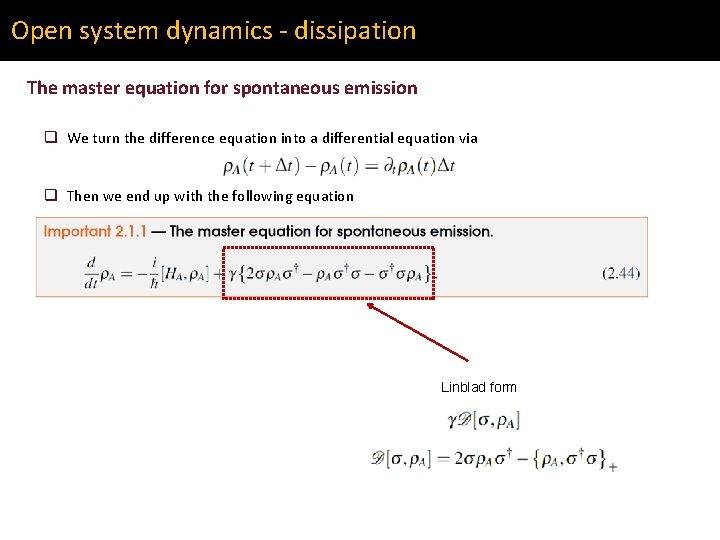
Open system dynamics - dissipation The master equation for spontaneous emission q We turn the difference equation into a differential equation via q Then we end up with the following equation Linblad form
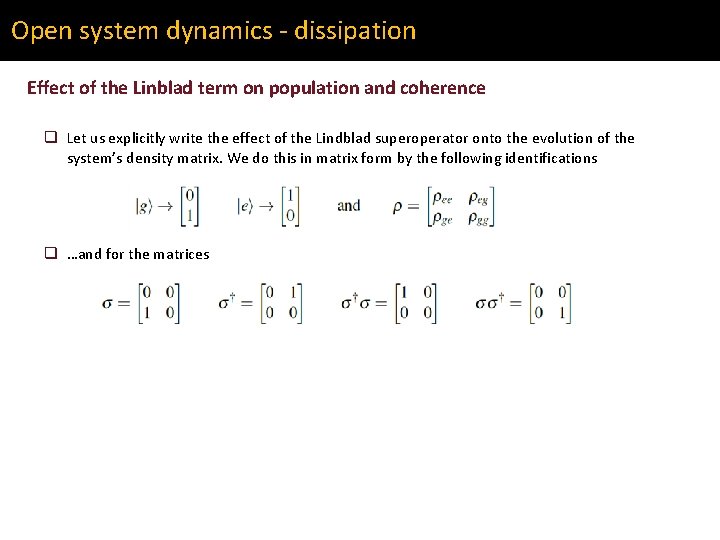
Open system dynamics - dissipation Effect of the Linblad term on population and coherence q Let us explicitly write the effect of the Lindblad superoperator onto the evolution of the system’s density matrix. We do this in matrix form by the following identifications q …and for the matrices
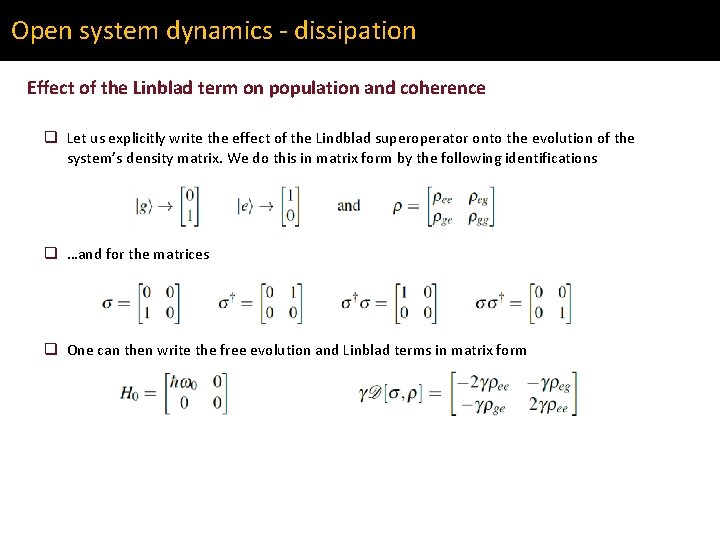
Open system dynamics - dissipation Effect of the Linblad term on population and coherence q Let us explicitly write the effect of the Lindblad superoperator onto the evolution of the system’s density matrix. We do this in matrix form by the following identifications q …and for the matrices q One can then write the free evolution and Linblad terms in matrix form
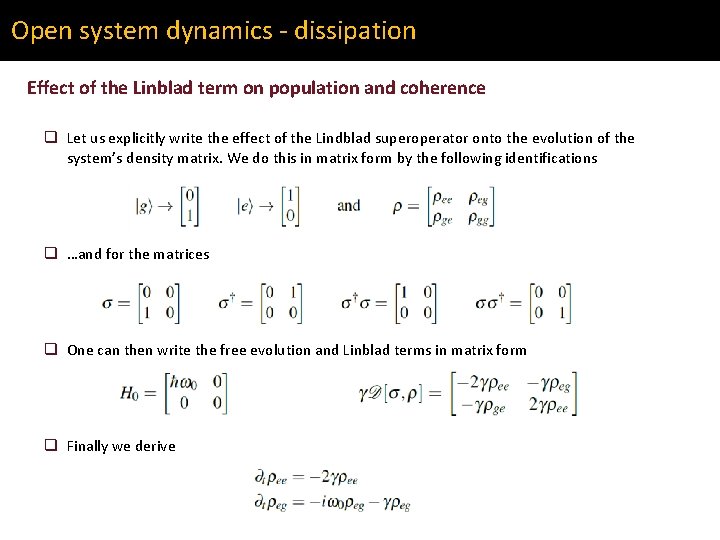
Open system dynamics - dissipation Effect of the Linblad term on population and coherence q Let us explicitly write the effect of the Lindblad superoperator onto the evolution of the system’s density matrix. We do this in matrix form by the following identifications q …and for the matrices q One can then write the free evolution and Linblad terms in matrix form q Finally we derive
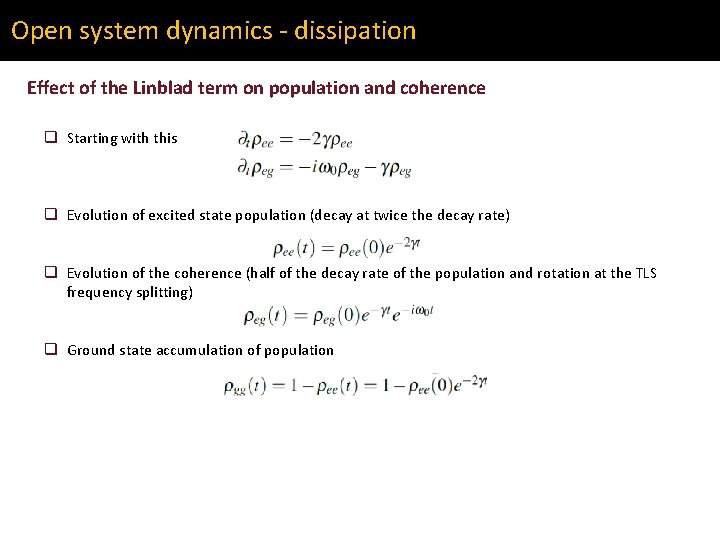
Open system dynamics - dissipation Effect of the Linblad term on population and coherence q Starting with this q Evolution of excited state population (decay at twice the decay rate) q Evolution of the coherence (half of the decay rate of the population and rotation at the TLS frequency splitting) q Ground state accumulation of population
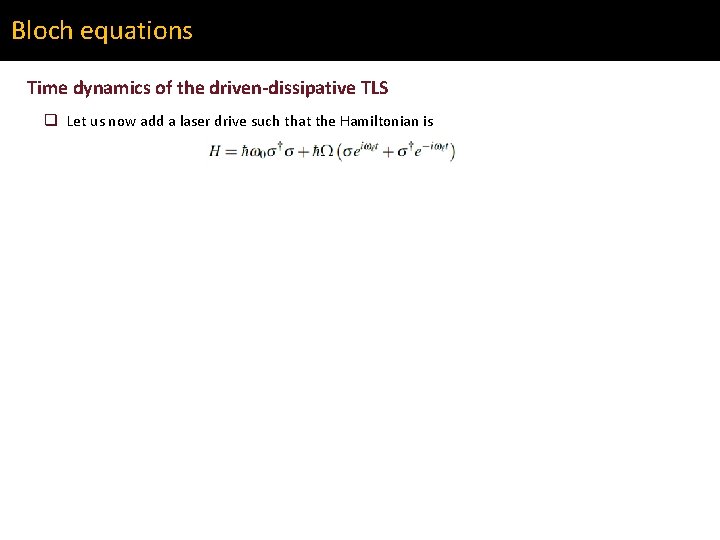
Bloch equations Time dynamics of the driven-dissipative TLS q Let us now add a laser drive such that the Hamiltonian is
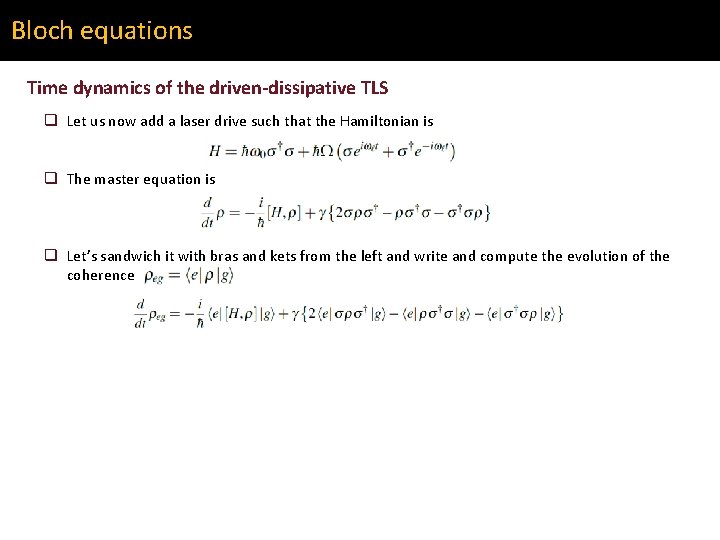
Bloch equations Time dynamics of the driven-dissipative TLS q Let us now add a laser drive such that the Hamiltonian is q The master equation is q Let’s sandwich it with bras and kets from the left and write and compute the evolution of the coherence
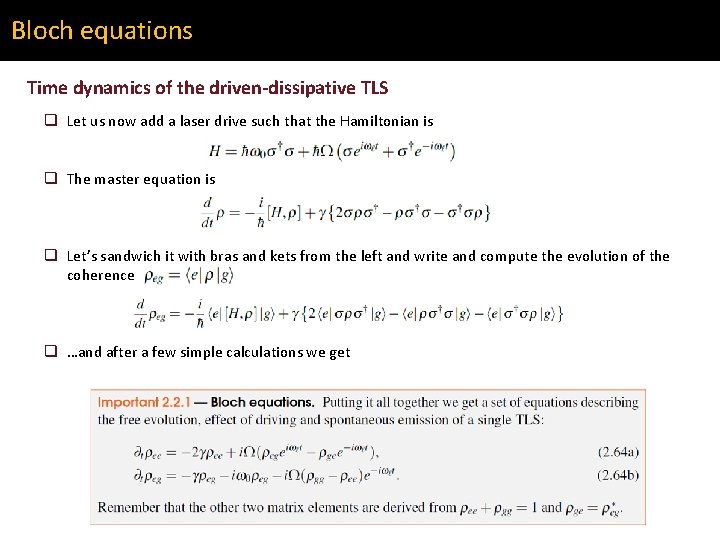
Bloch equations Time dynamics of the driven-dissipative TLS q Let us now add a laser drive such that the Hamiltonian is q The master equation is q Let’s sandwich it with bras and kets from the left and write and compute the evolution of the coherence q …and after a few simple calculations we get
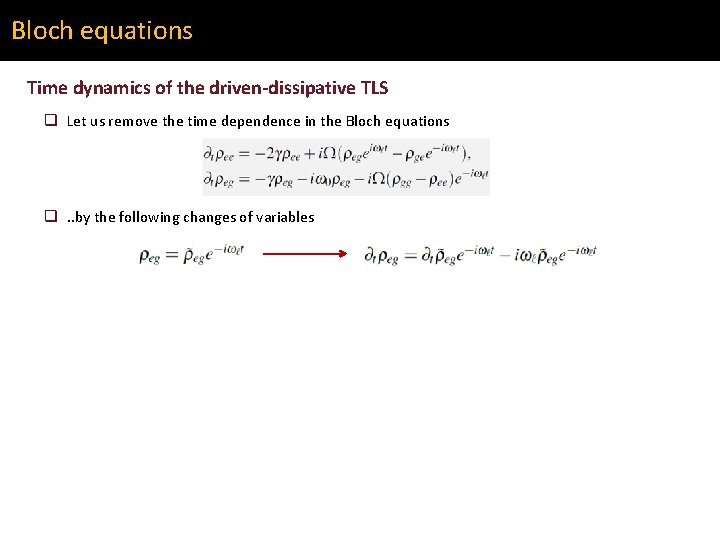
Bloch equations Time dynamics of the driven-dissipative TLS q Let us remove the time dependence in the Bloch equations q. . by the following changes of variables
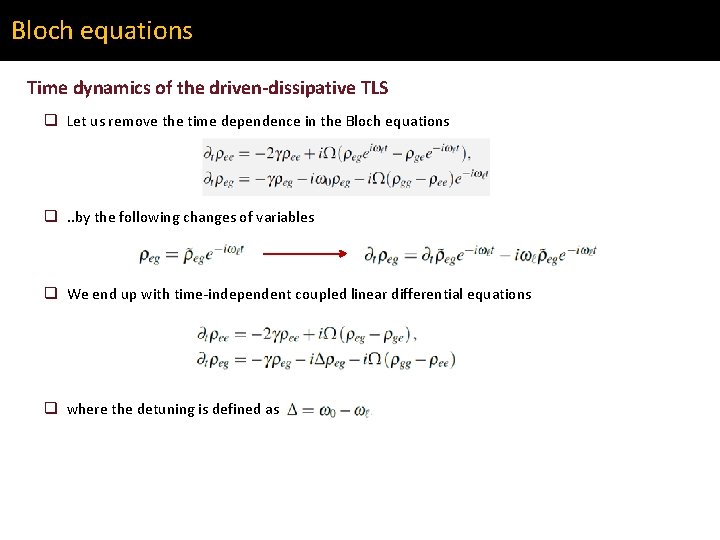
Bloch equations Time dynamics of the driven-dissipative TLS q Let us remove the time dependence in the Bloch equations q. . by the following changes of variables q We end up with time-independent coupled linear differential equations q where the detuning is defined as
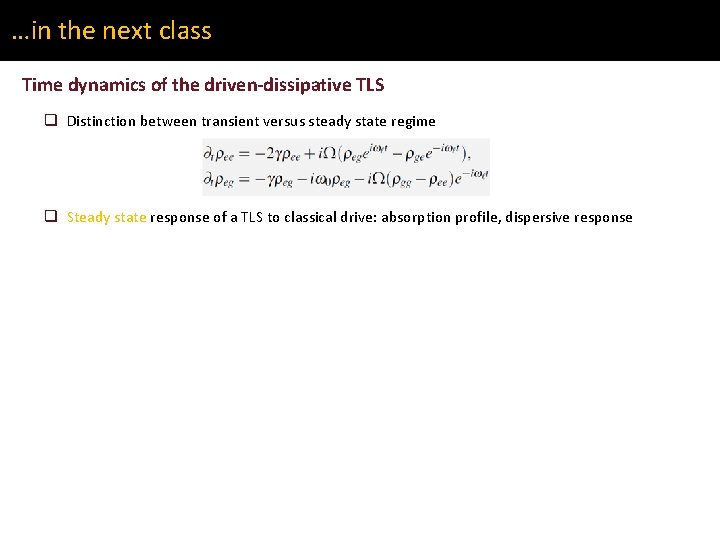
…in the next class Time dynamics of the driven-dissipative TLS q Distinction between transient versus steady state regime q Steady state response of a TLS to classical drive: absorption profile, dispersive response
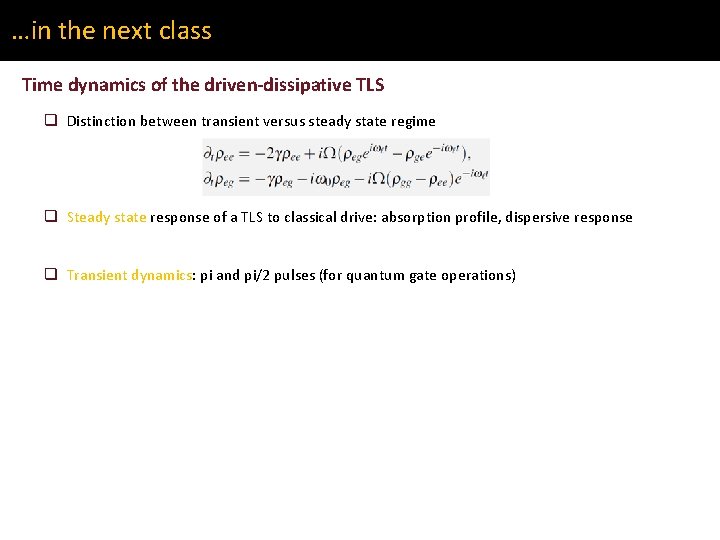
…in the next class Time dynamics of the driven-dissipative TLS q Distinction between transient versus steady state regime q Steady state response of a TLS to classical drive: absorption profile, dispersive response q Transient dynamics: pi and pi/2 pulses (for quantum gate operations)
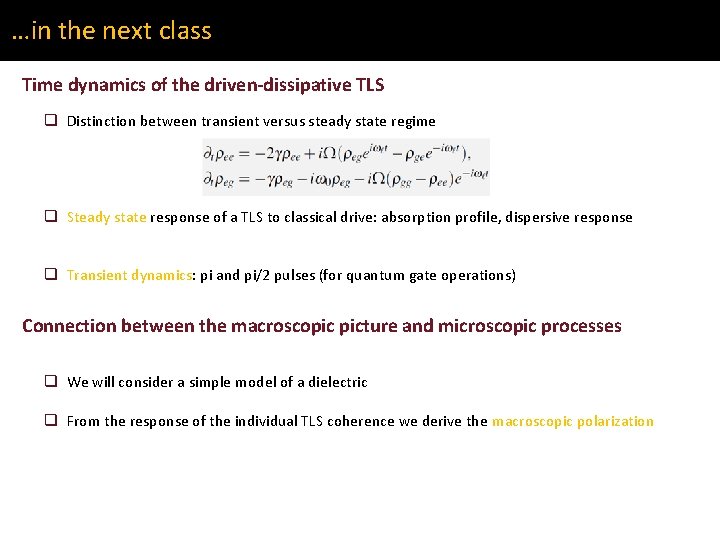
…in the next class Time dynamics of the driven-dissipative TLS q Distinction between transient versus steady state regime q Steady state response of a TLS to classical drive: absorption profile, dispersive response q Transient dynamics: pi and pi/2 pulses (for quantum gate operations) Connection between the macroscopic picture and microscopic processes q We will consider a simple model of a dielectric q From the response of the individual TLS coherence we derive the macroscopic polarization
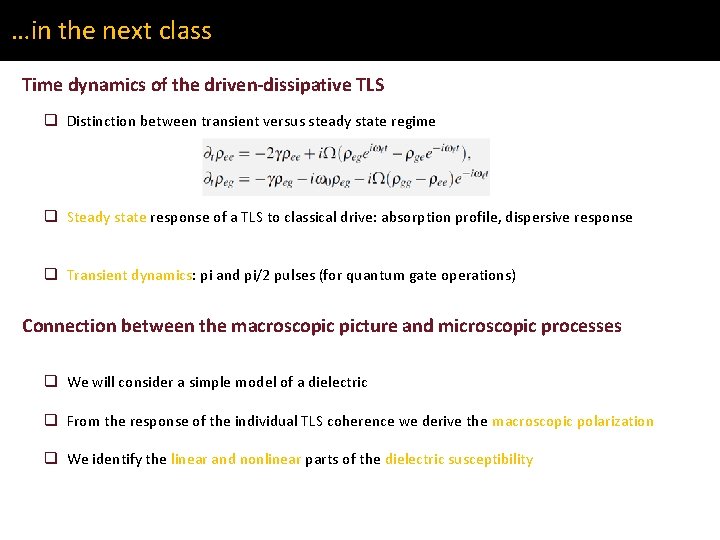
…in the next class Time dynamics of the driven-dissipative TLS q Distinction between transient versus steady state regime q Steady state response of a TLS to classical drive: absorption profile, dispersive response q Transient dynamics: pi and pi/2 pulses (for quantum gate operations) Connection between the macroscopic picture and microscopic processes q We will consider a simple model of a dielectric q From the response of the individual TLS coherence we derive the macroscopic polarization q We identify the linear and nonlinear parts of the dielectric susceptibility
Classical mechanics
Quantum physics vs quantum mechanics
Beta positive decay
Seth aubin
Physics topic 12
Quantum physics wave function
Electron volt to volt
Quantum physics wave function
Review of quantum mechanics
Quantum physics in hindi
Khan academy pn junction
Quantum nuclear physics
Why does it happen
University physics with modern physics fifteenth edition
Physics ia rubric
Axial substituents
Nervous interactions with other systems
Interactions in the environment grade 7
Interactions between atoms occur
5 major types of species interactions
Types of parasitism
Synergism
Unit 5 ecology
Pathway of food through digestive tract
Types of interactions
Project management process interactions
The mimosa plant displays thigmotropism
Intermolecular force
Gene interactions
The maximum distance a wave varies from its rest position
Graphing monetary and fiscal policy interactions
Foss chemical interactions
Chapter 22 reaching out cross-cultural interactions
Integral product architecture
Interactions
Diazepam cyp450
Skeletal interactions with other systems
What is abiotic factor
Erleada drug interactions
Joseph connell barnacle experiment
Ecosystems interactions
Heterotrophs
Naive bayes pays attention to complex interactions and
Circulatory system interactions with other systems
Wave interactions
Niches biology
The properties and interactions of magnets
Regional and transregional interactions
Insight therapies involve verbal interactions
Noncovalent interactions
Niches and community interactions
Chapter 22 reaching out cross-cultural interactions
Special interactions
Gene interactions
Discuss some factors influencing communication