Polymers with special interactions Polymers actually copolymers may
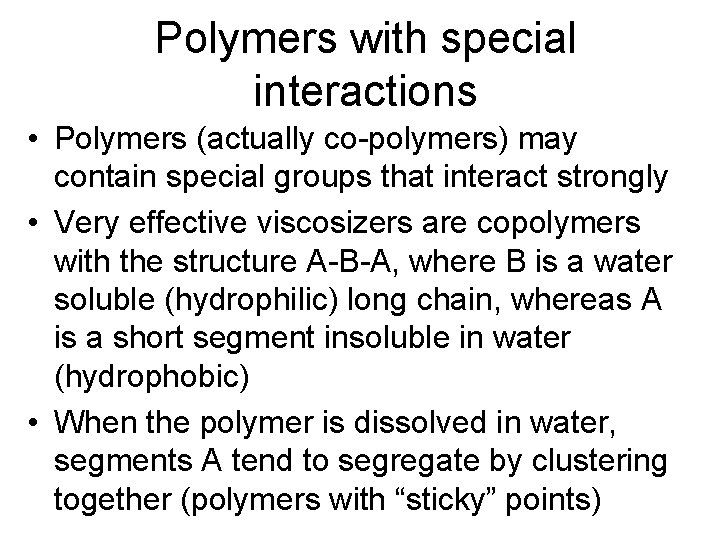
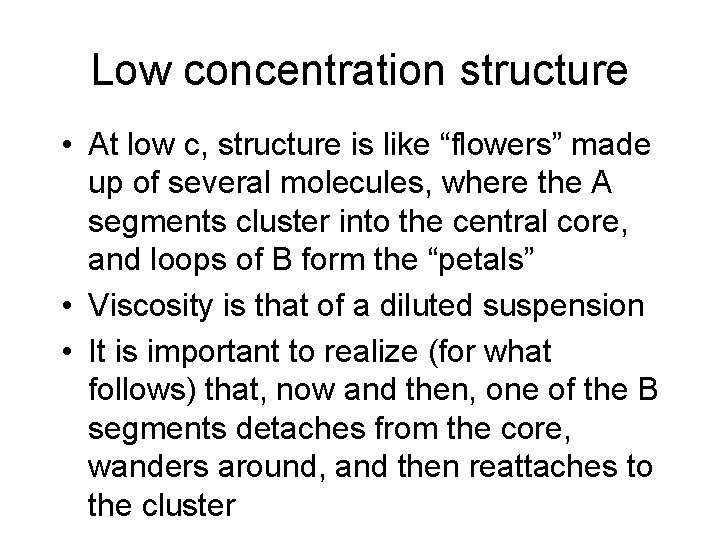
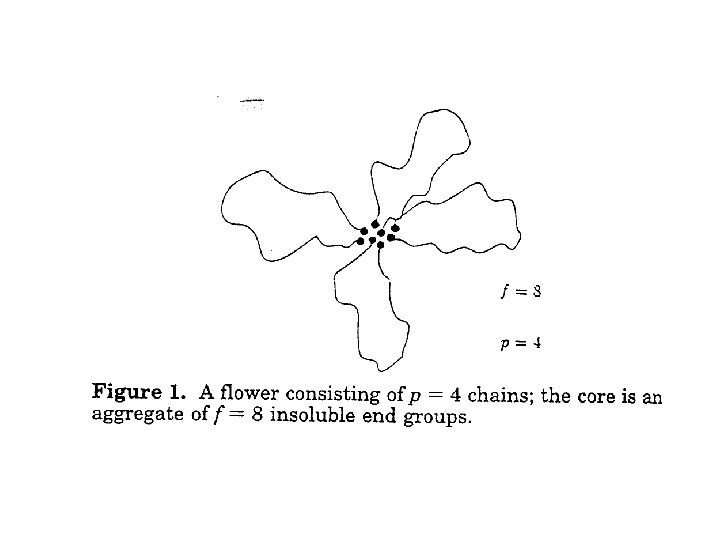
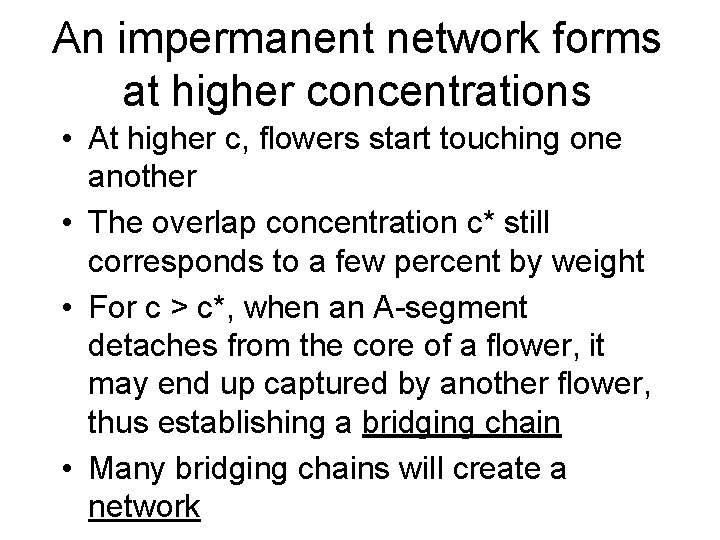
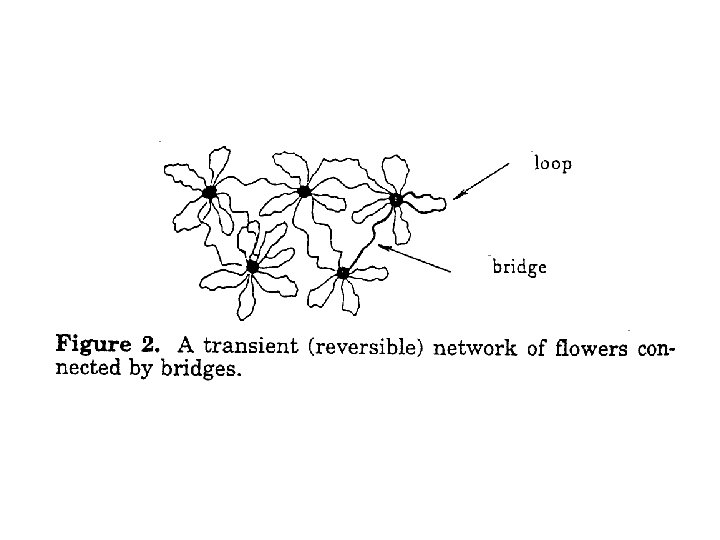
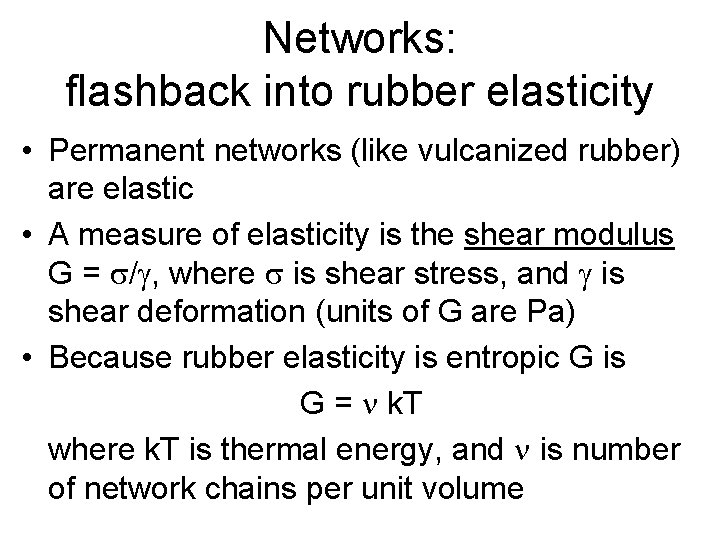
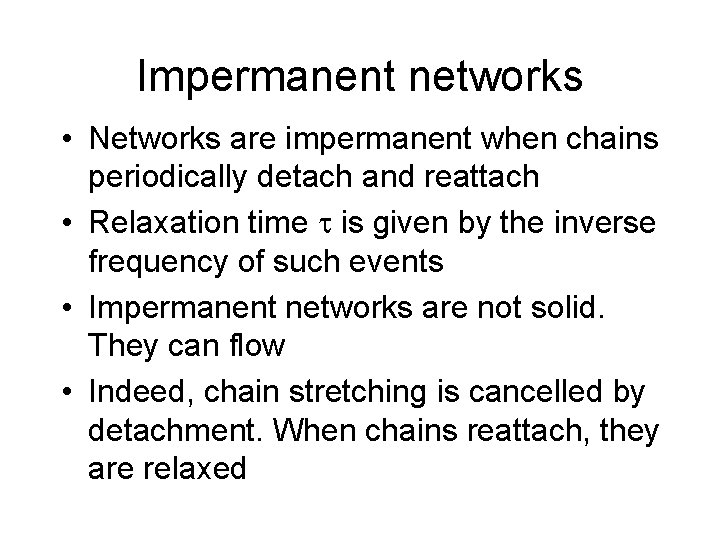
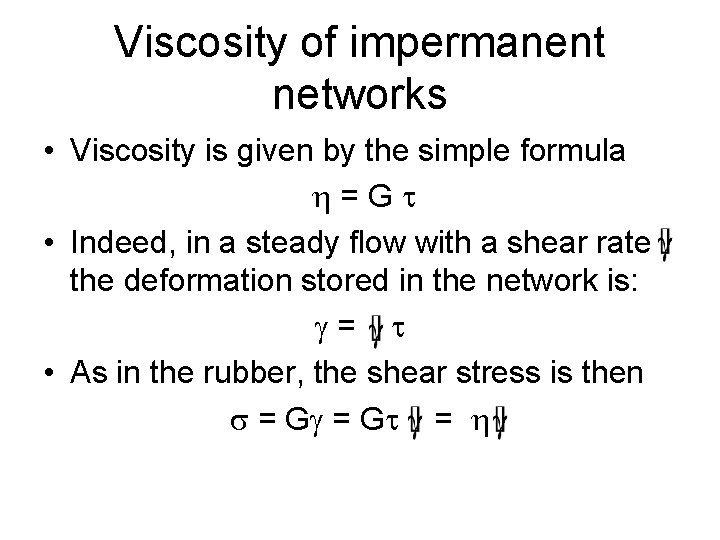
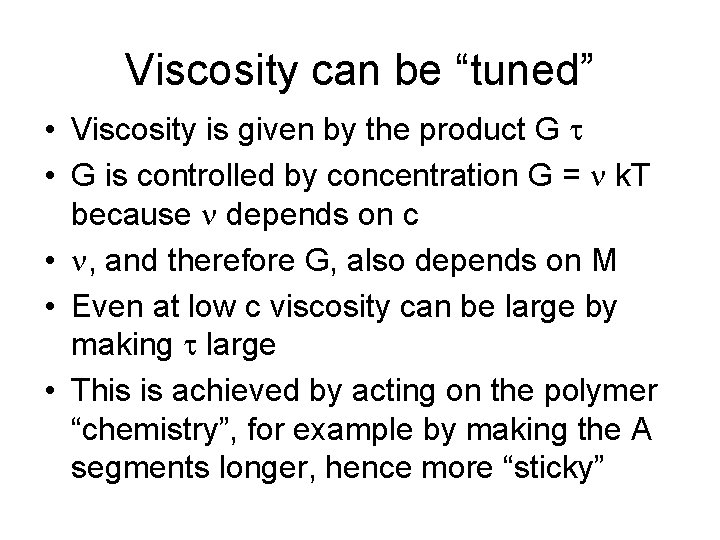
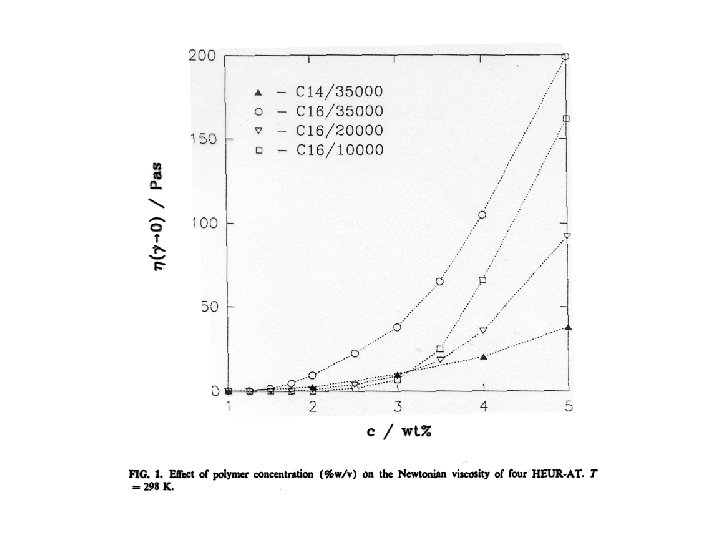
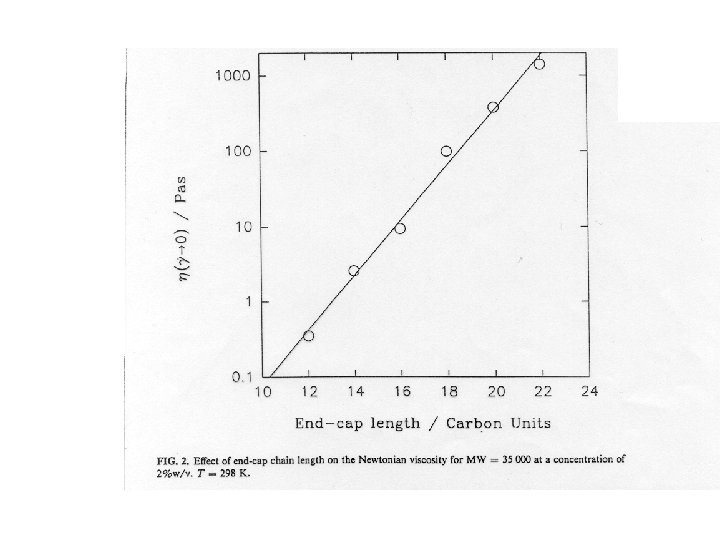
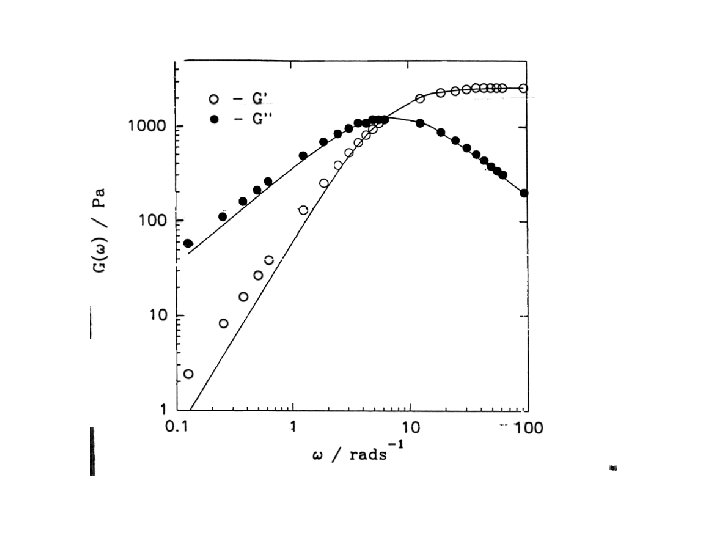
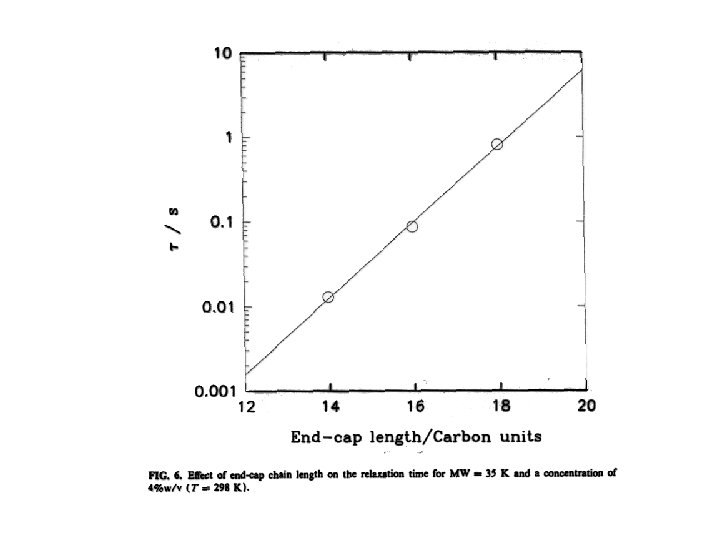
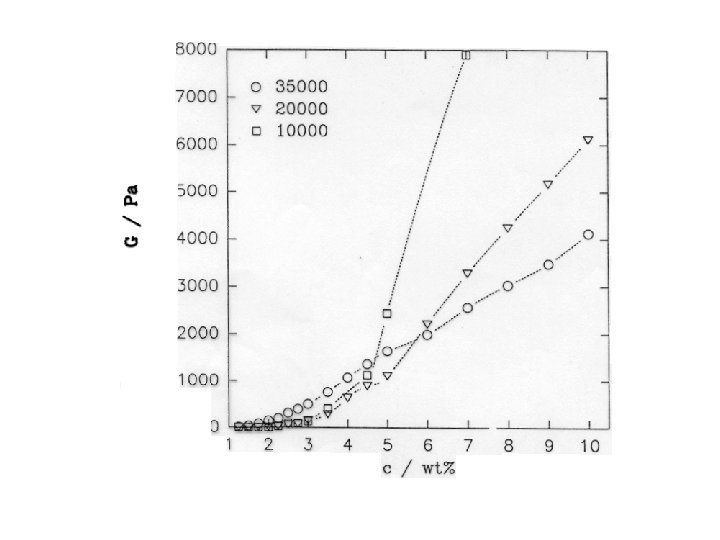
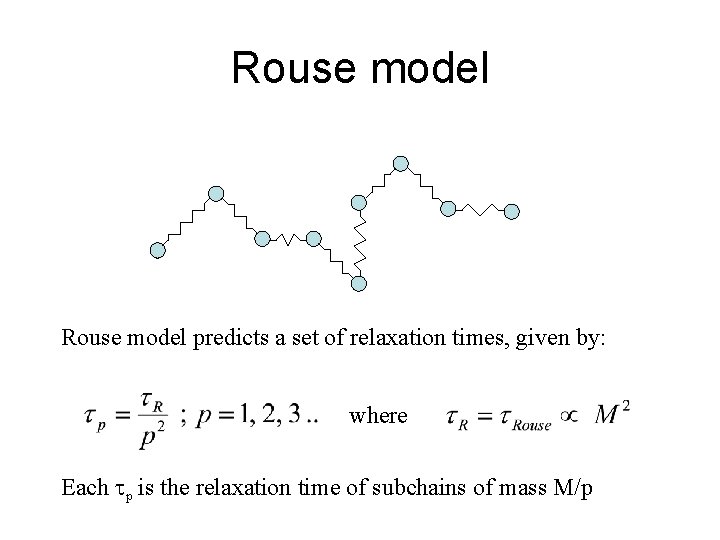
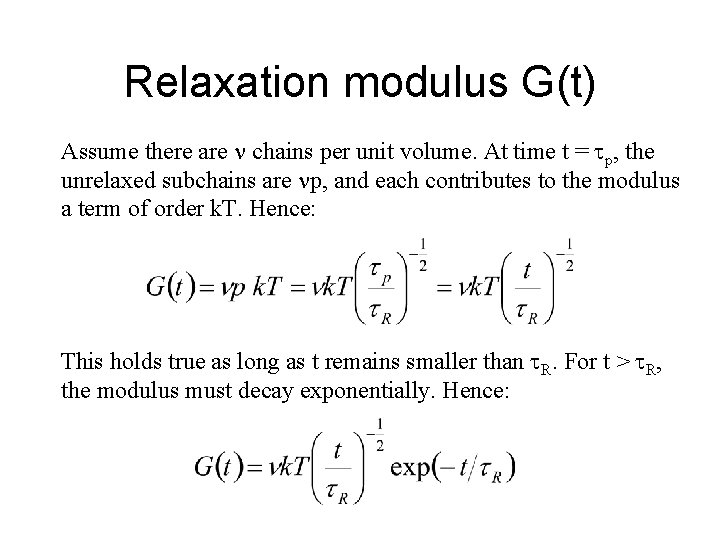
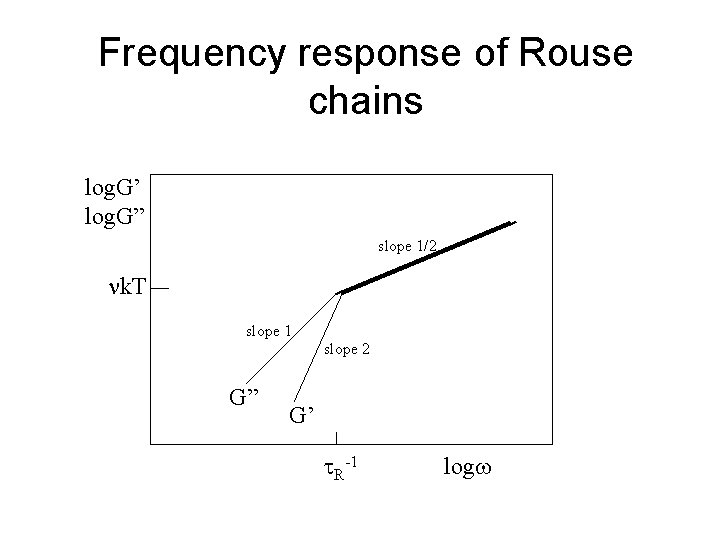
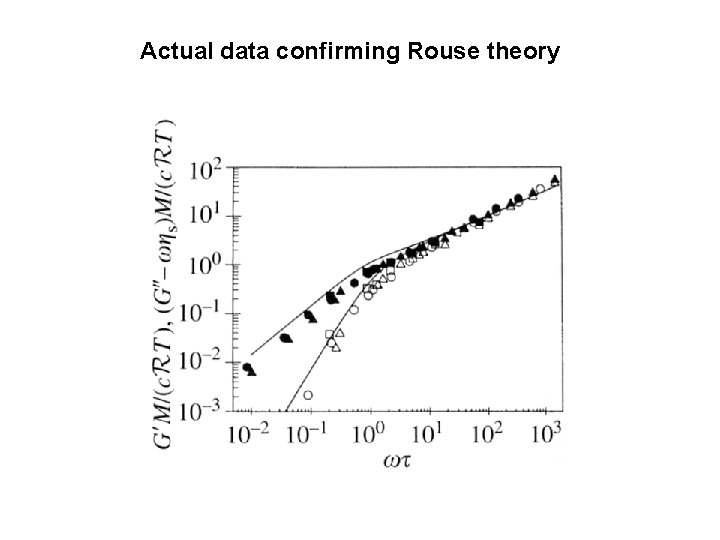
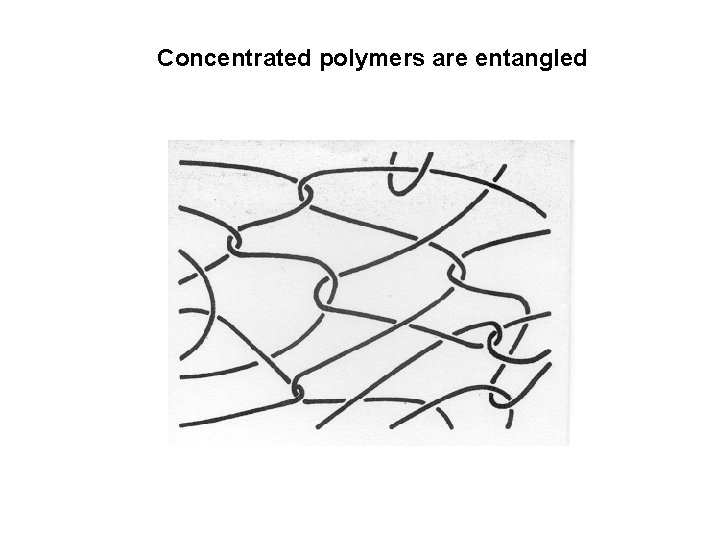
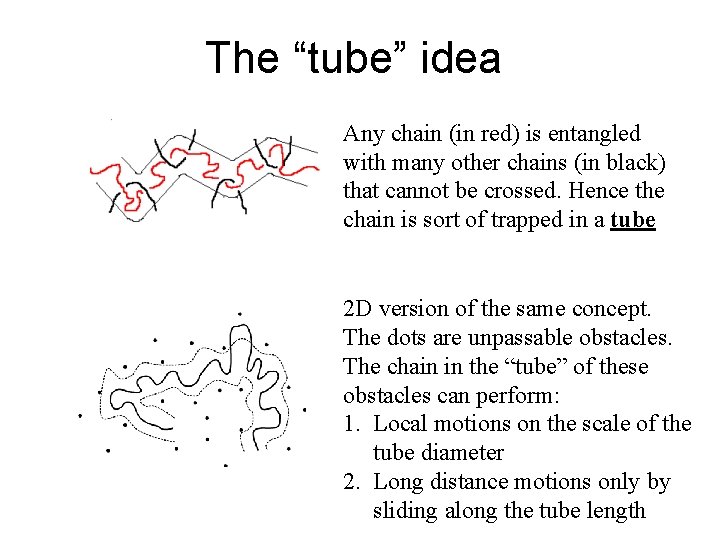
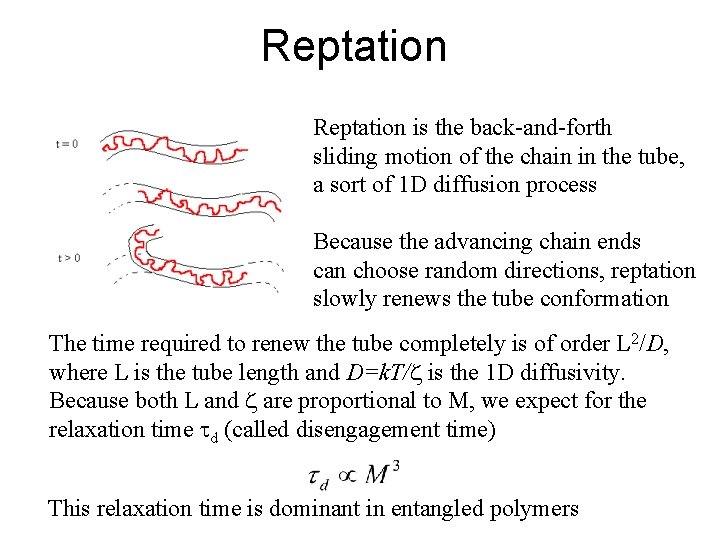
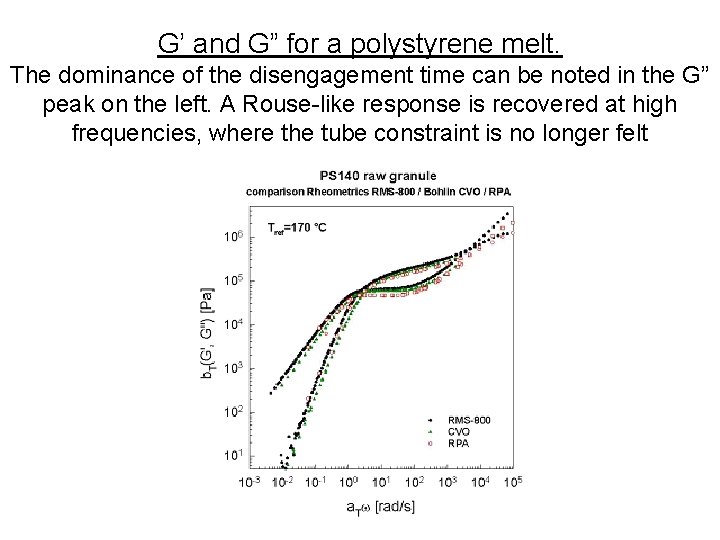
- Slides: 22
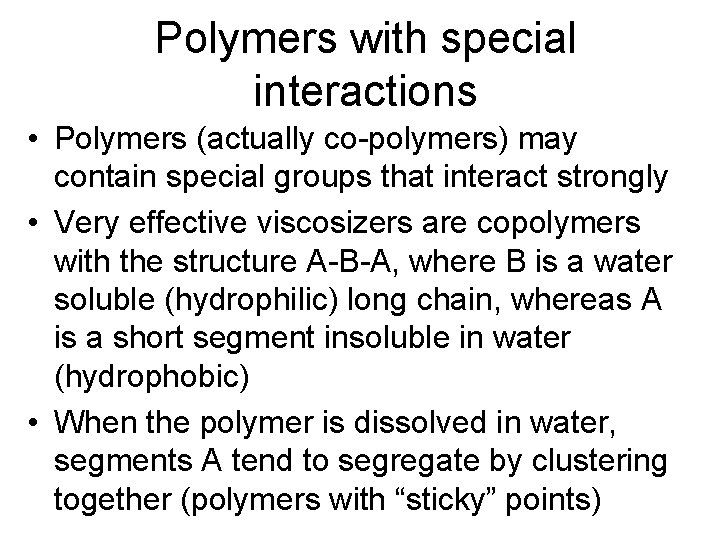
Polymers with special interactions • Polymers (actually co-polymers) may contain special groups that interact strongly • Very effective viscosizers are copolymers with the structure A-B-A, where B is a water soluble (hydrophilic) long chain, whereas A is a short segment insoluble in water (hydrophobic) • When the polymer is dissolved in water, segments A tend to segregate by clustering together (polymers with “sticky” points)
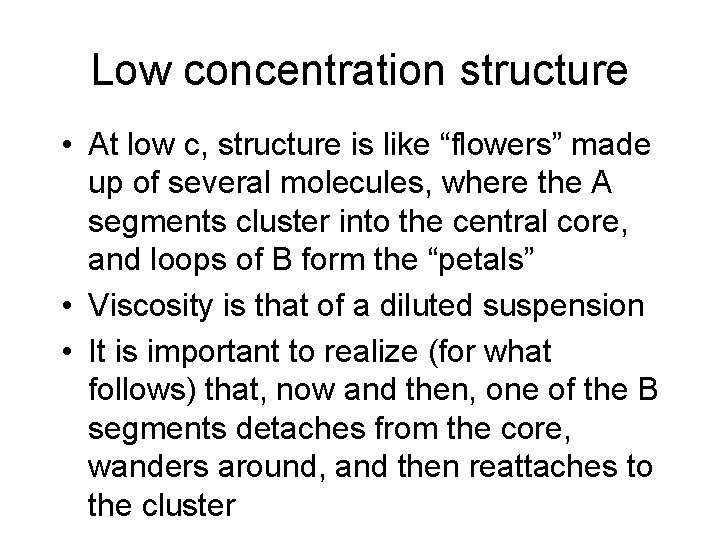
Low concentration structure • At low c, structure is like “flowers” made up of several molecules, where the A segments cluster into the central core, and loops of B form the “petals” • Viscosity is that of a diluted suspension • It is important to realize (for what follows) that, now and then, one of the B segments detaches from the core, wanders around, and then reattaches to the cluster
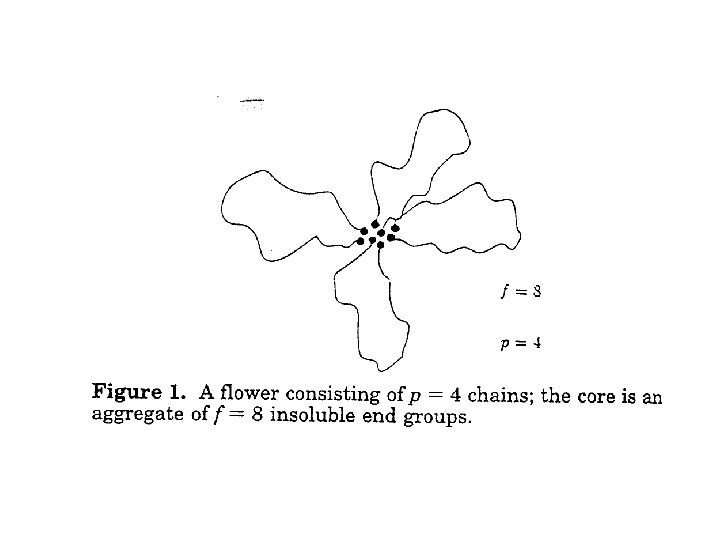
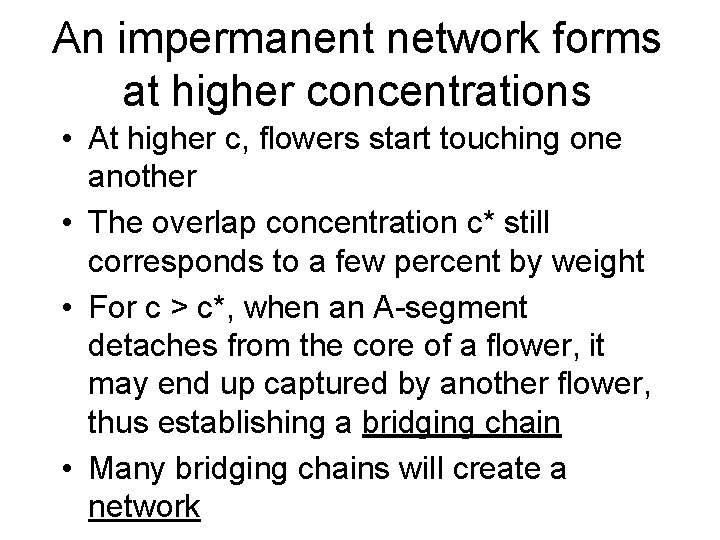
An impermanent network forms at higher concentrations • At higher c, flowers start touching one another • The overlap concentration c* still corresponds to a few percent by weight • For c > c*, when an A-segment detaches from the core of a flower, it may end up captured by another flower, thus establishing a bridging chain • Many bridging chains will create a network
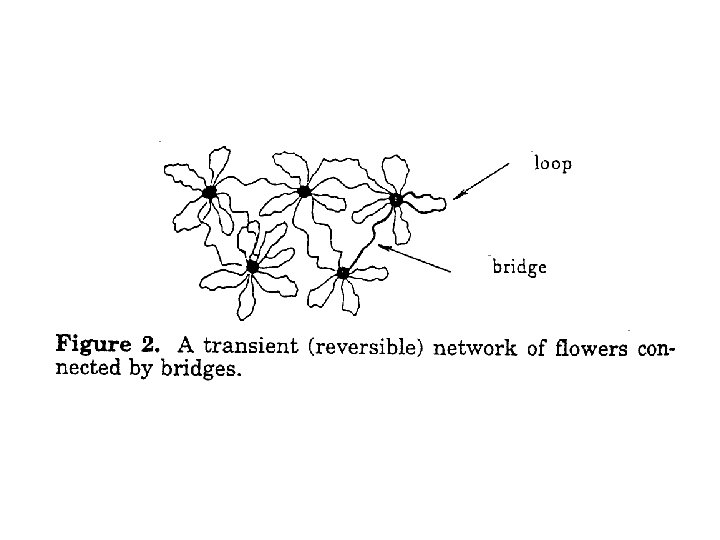
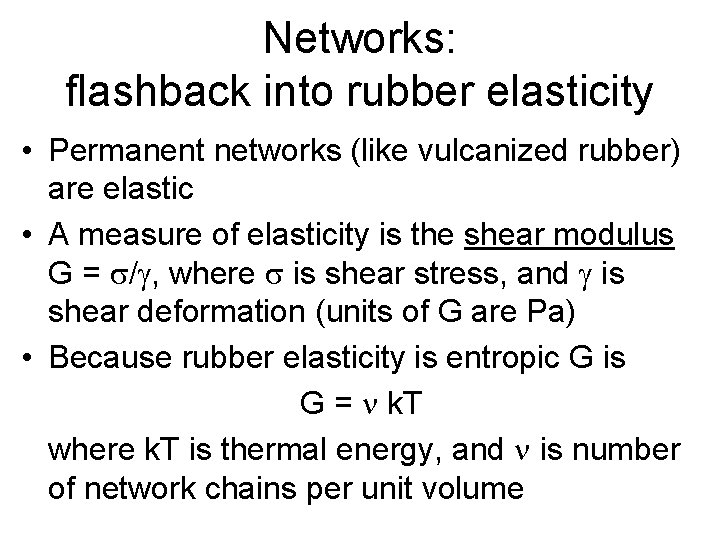
Networks: flashback into rubber elasticity • Permanent networks (like vulcanized rubber) are elastic • A measure of elasticity is the shear modulus G = / , where is shear stress, and is shear deformation (units of G are Pa) • Because rubber elasticity is entropic G is G = k. T where k. T is thermal energy, and is number of network chains per unit volume
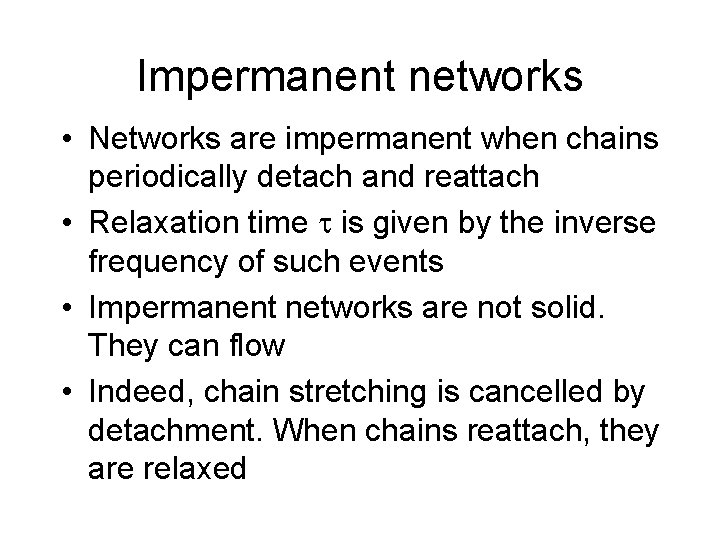
Impermanent networks • Networks are impermanent when chains periodically detach and reattach • Relaxation time is given by the inverse frequency of such events • Impermanent networks are not solid. They can flow • Indeed, chain stretching is cancelled by detachment. When chains reattach, they are relaxed
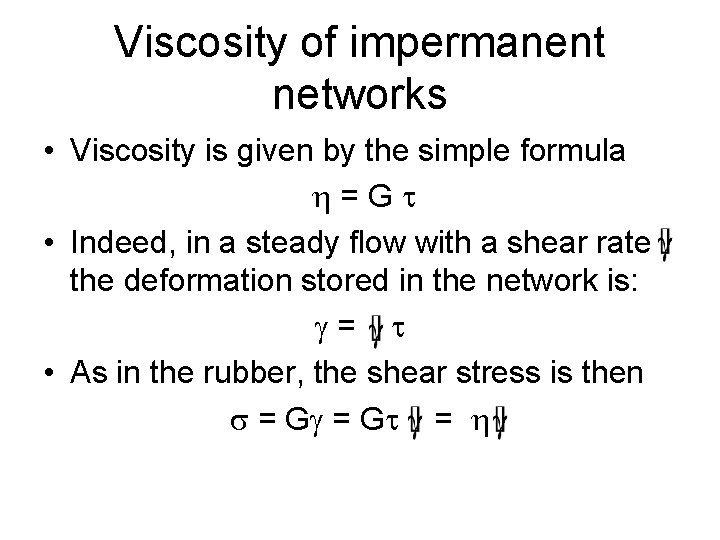
Viscosity of impermanent networks • Viscosity is given by the simple formula = G • Indeed, in a steady flow with a shear rate the deformation stored in the network is: = • As in the rubber, the shear stress is then = G =
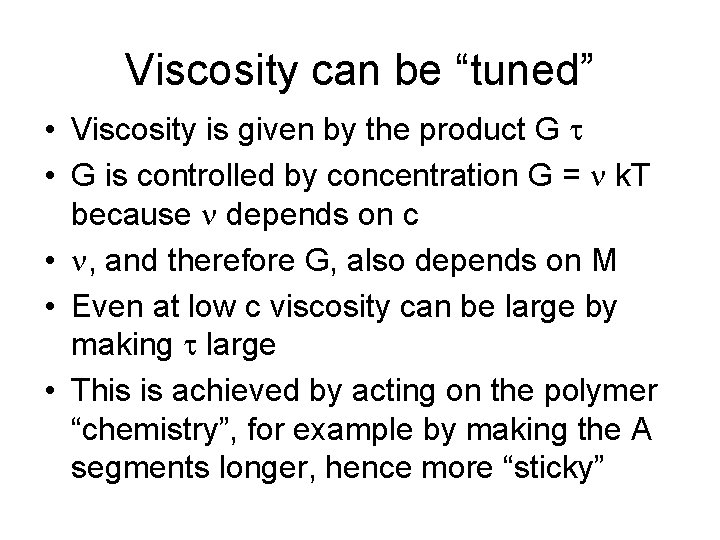
Viscosity can be “tuned” • Viscosity is given by the product G • G is controlled by concentration G = k. T because depends on c • , and therefore G, also depends on M • Even at low c viscosity can be large by making large • This is achieved by acting on the polymer “chemistry”, for example by making the A segments longer, hence more “sticky”
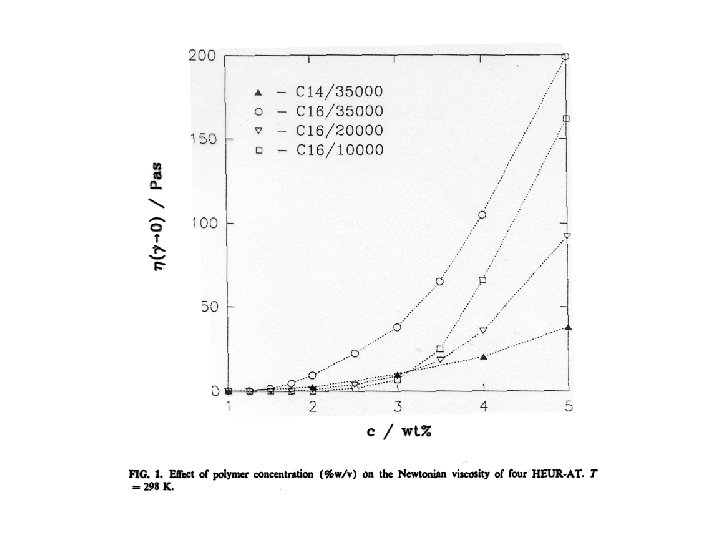
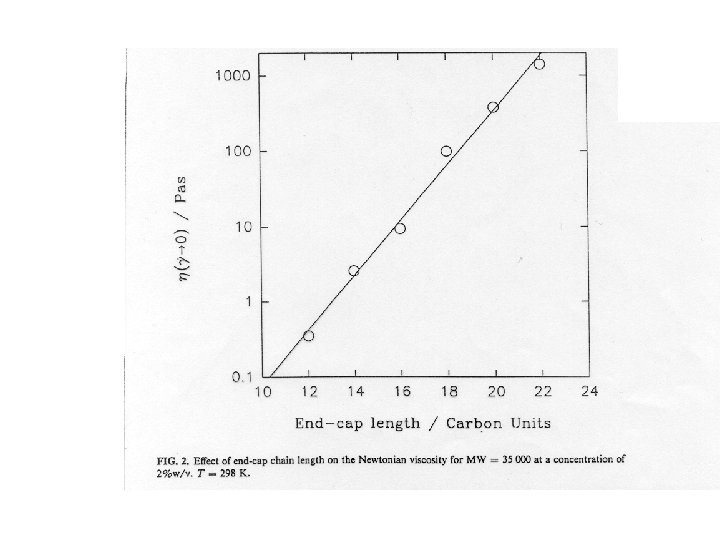
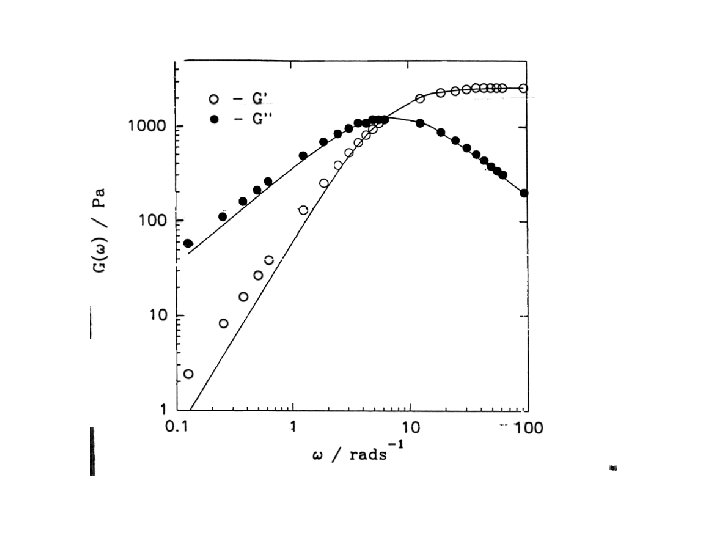
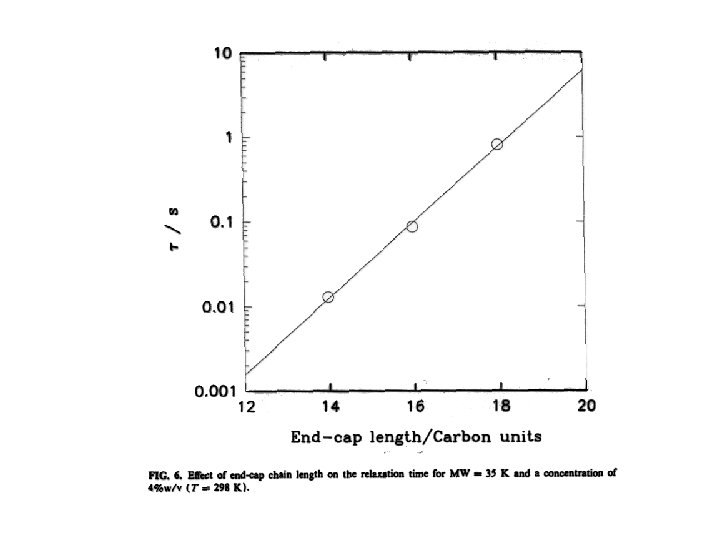
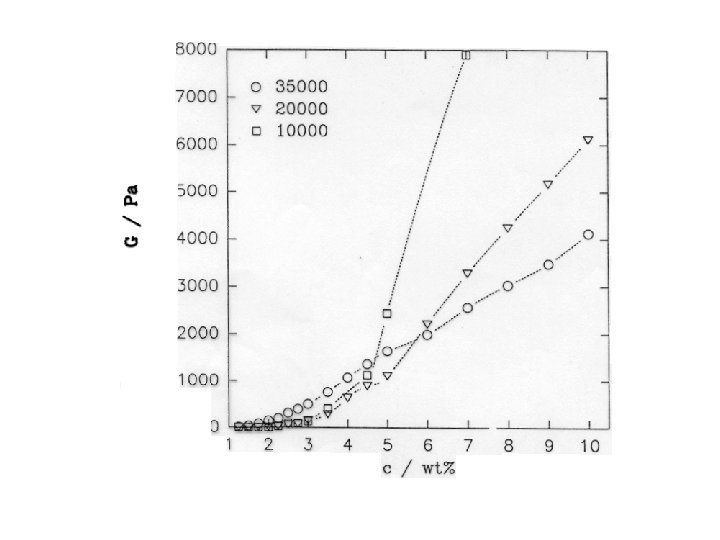
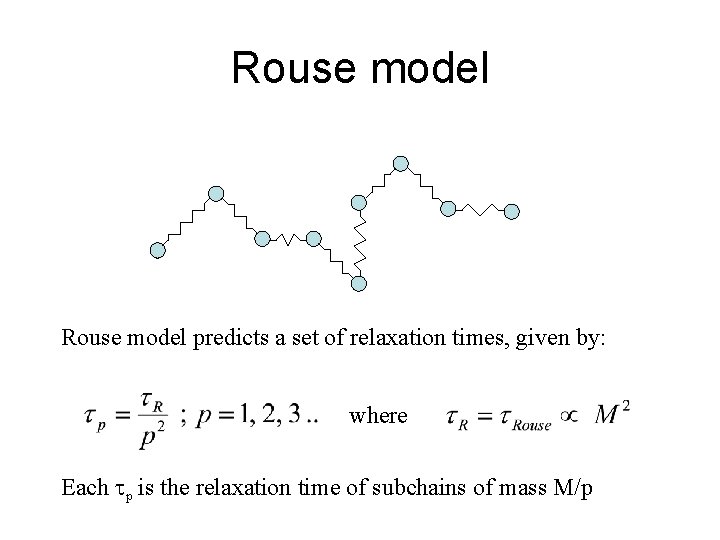
Rouse model predicts a set of relaxation times, given by: where Each p is the relaxation time of subchains of mass M/p
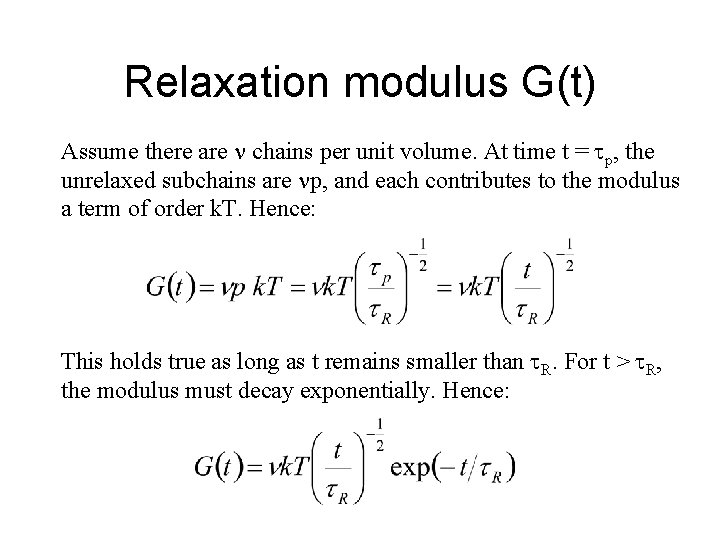
Relaxation modulus G(t) Assume there are chains per unit volume. At time t = p, the unrelaxed subchains are p, and each contributes to the modulus a term of order k. T. Hence: This holds true as long as t remains smaller than R. For t > R, the modulus must decay exponentially. Hence:
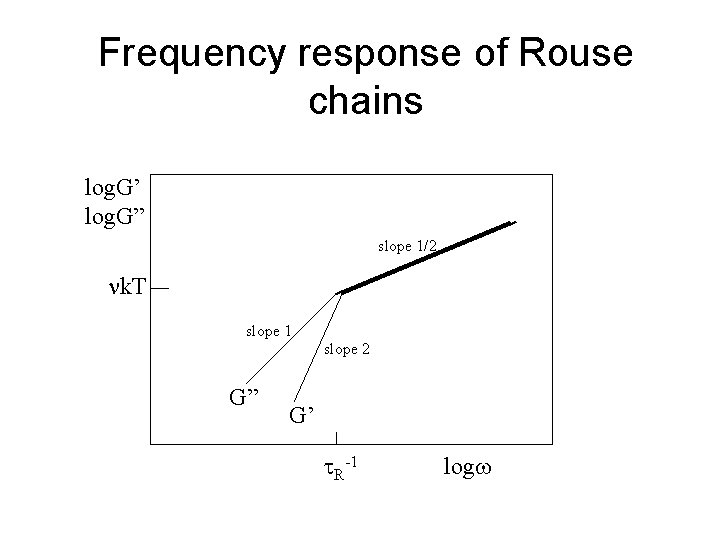
Frequency response of Rouse chains log. G’ log. G” slope 1/2 k. T slope 1 slope 2 G” G’ R-1 logw
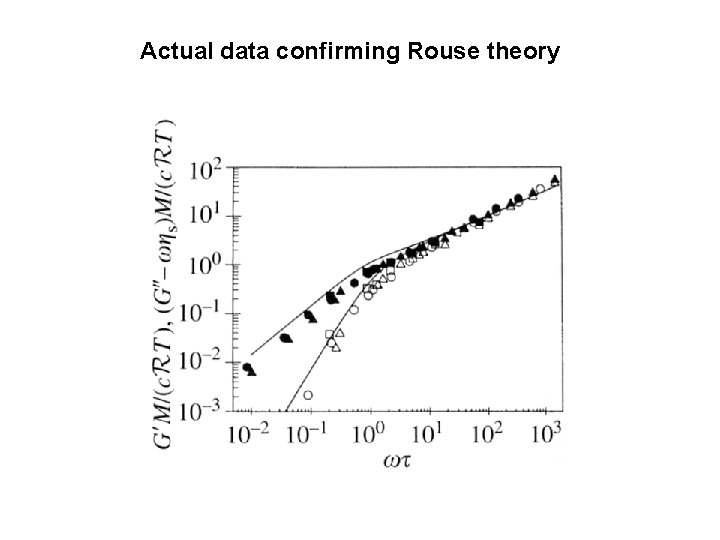
Actual data confirming Rouse theory
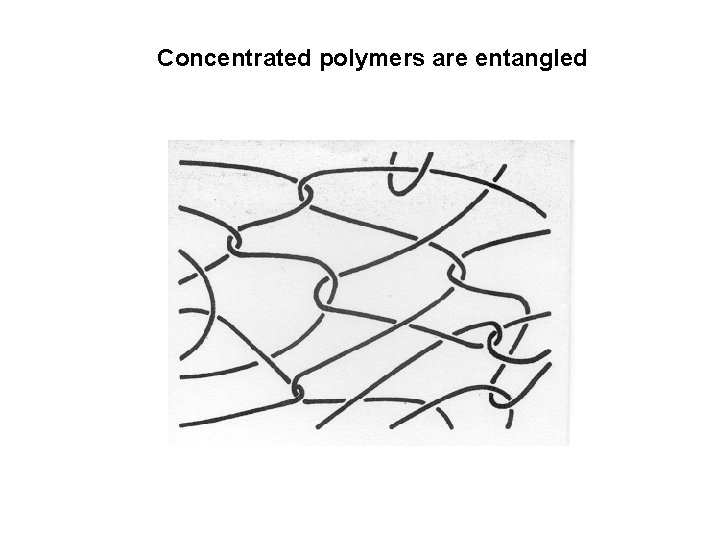
Concentrated polymers are entangled
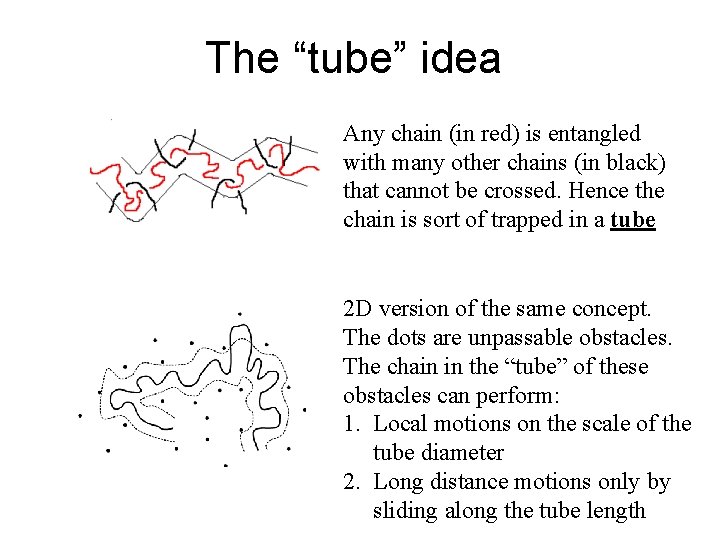
The “tube” idea Any chain (in red) is entangled with many other chains (in black) that cannot be crossed. Hence the chain is sort of trapped in a tube 2 D version of the same concept. The dots are unpassable obstacles. The chain in the “tube” of these obstacles can perform: 1. Local motions on the scale of the tube diameter 2. Long distance motions only by sliding along the tube length
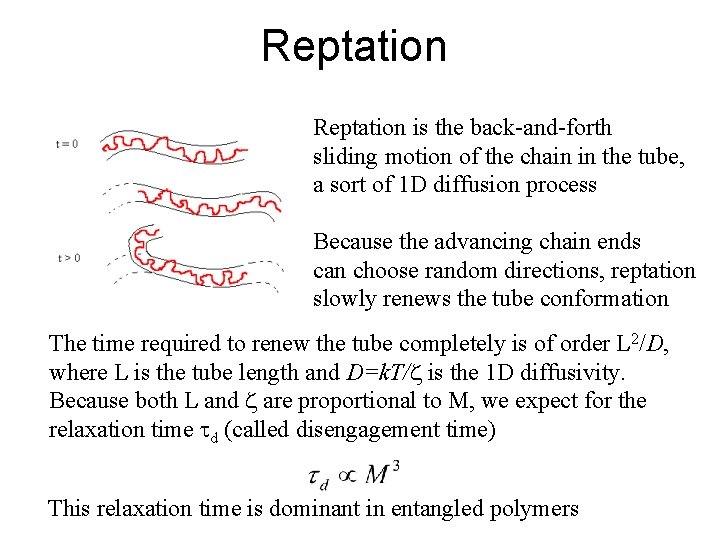
Reptation is the back-and-forth sliding motion of the chain in the tube, a sort of 1 D diffusion process Because the advancing chain ends can choose random directions, reptation slowly renews the tube conformation The time required to renew the tube completely is of order L 2/D, where L is the tube length and D=k. T/z is the 1 D diffusivity. Because both L and z are proportional to M, we expect for the relaxation time d (called disengagement time) This relaxation time is dominant in entangled polymers
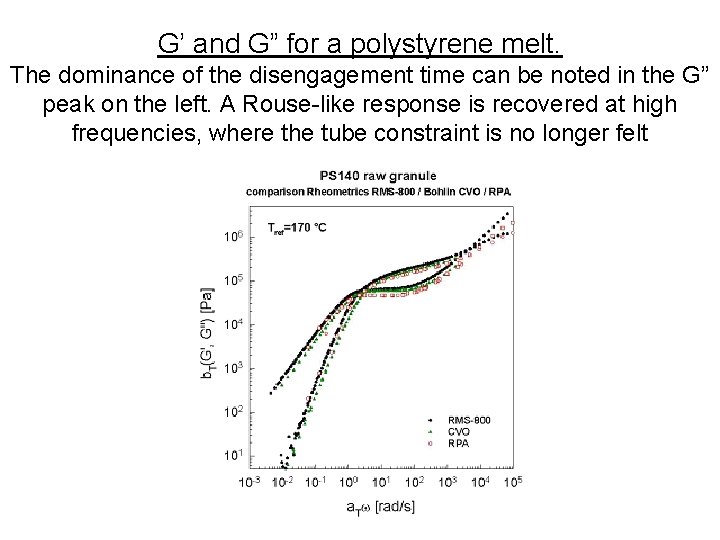
G’ and G” for a polystyrene melt. The dominance of the disengagement time can be noted in the G” peak on the left. A Rouse-like response is recovered at high frequencies, where the tube constraint is no longer felt
Chm138
Condensation copolymers
Special interactions
The elements which you have actually seen
Measure synoynm
Scalp care shampooing and conditioning
Critical thinking definition
What is logic
Real self and ideal self examples
Wind energy is an indirect form of
Great gatsby ch 8 summary
The contrast between what happens and what was expected
Actually that was america
The inhalant nitrous oxide can rob the body of
Hci design patterns
Hccee
Regional and transregional interactions
Naive bayes pays attention to complex interactions and
Interactions among living things
6.1 habitats niches and species interactions
Circulatory system interactions with other systems
Integumentary interactions with other systems
The properties and interactions of magnets