Prospects for the induction acceleration in FFAGs Neil
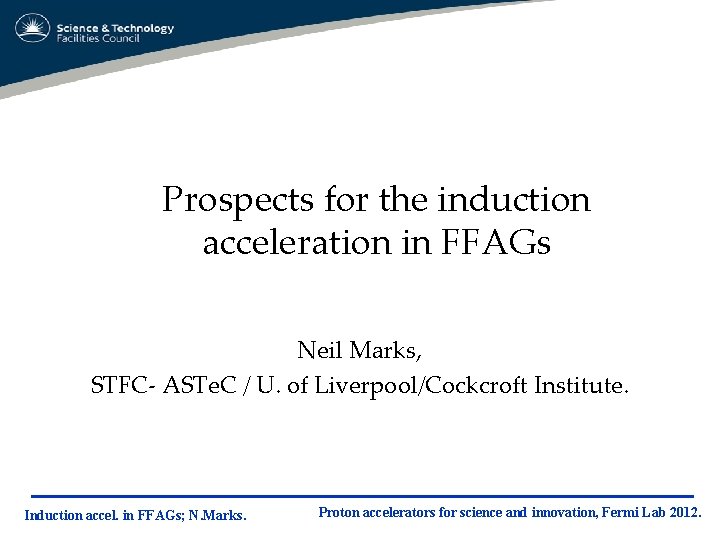
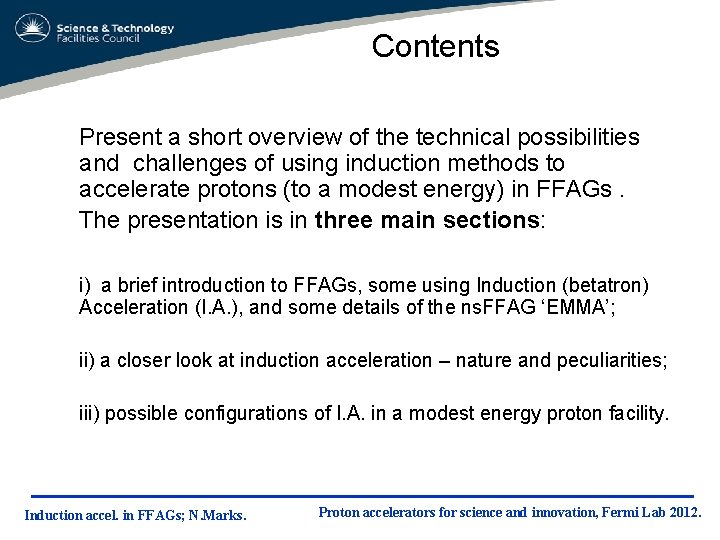
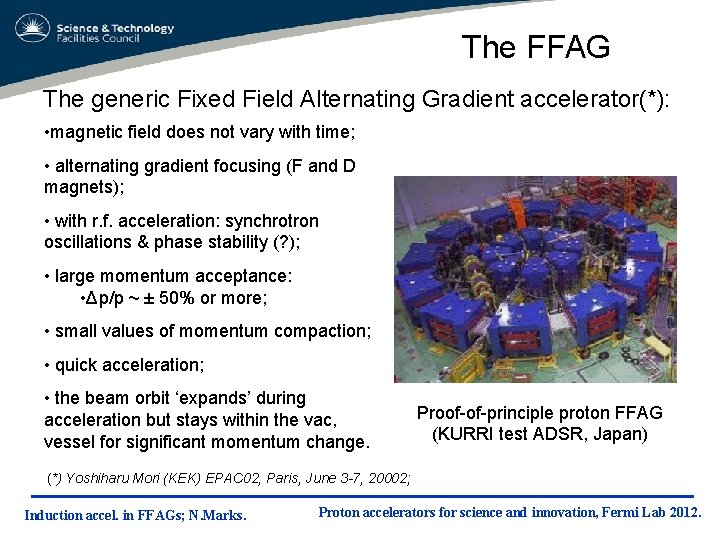
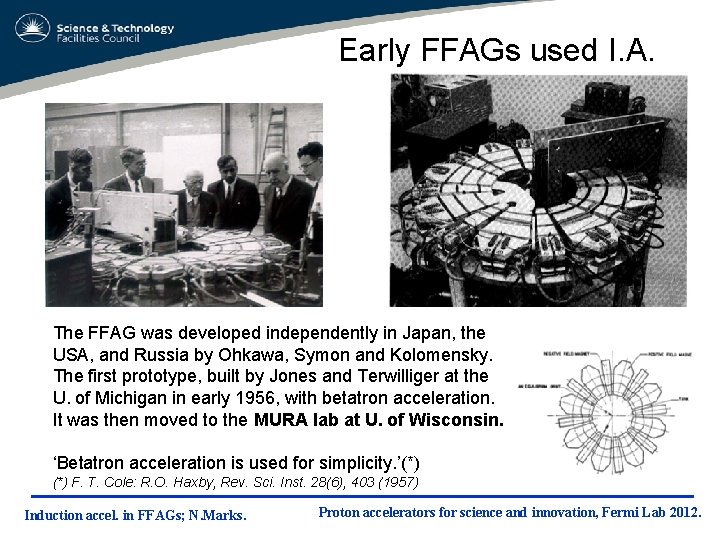
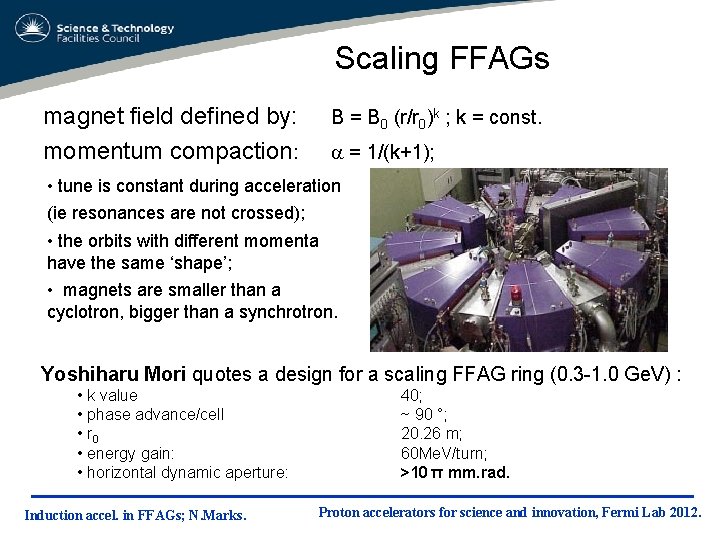
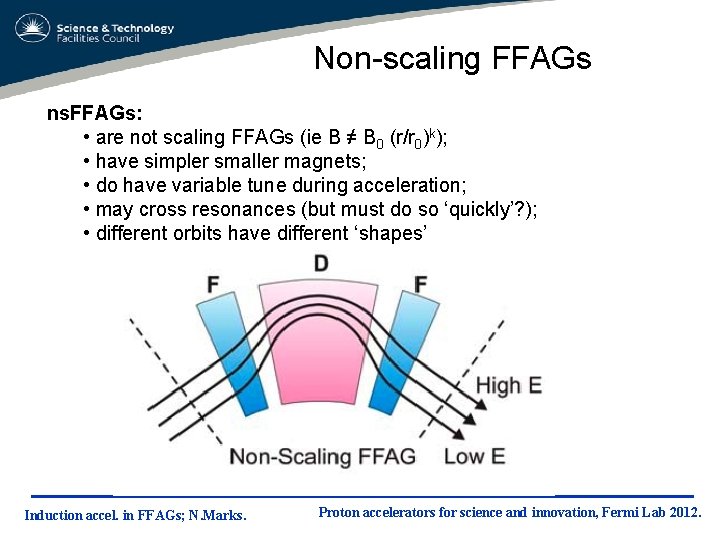
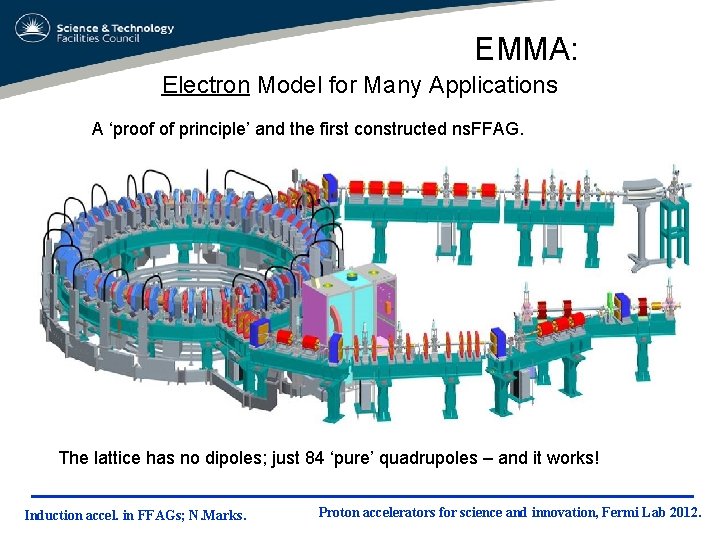
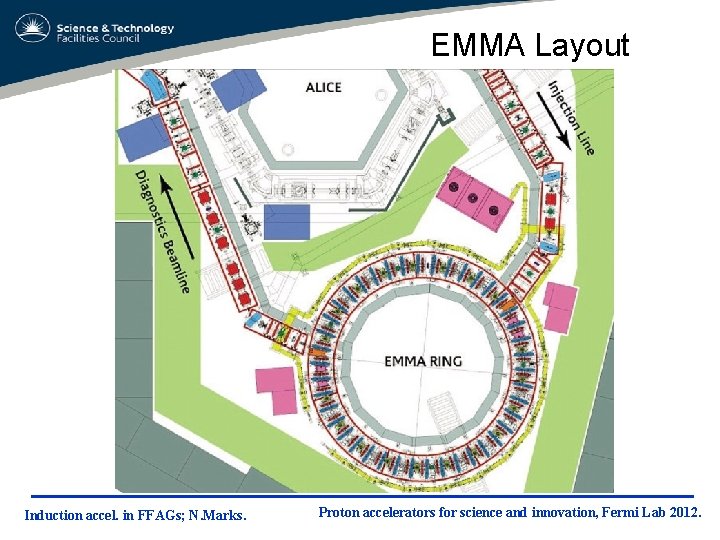
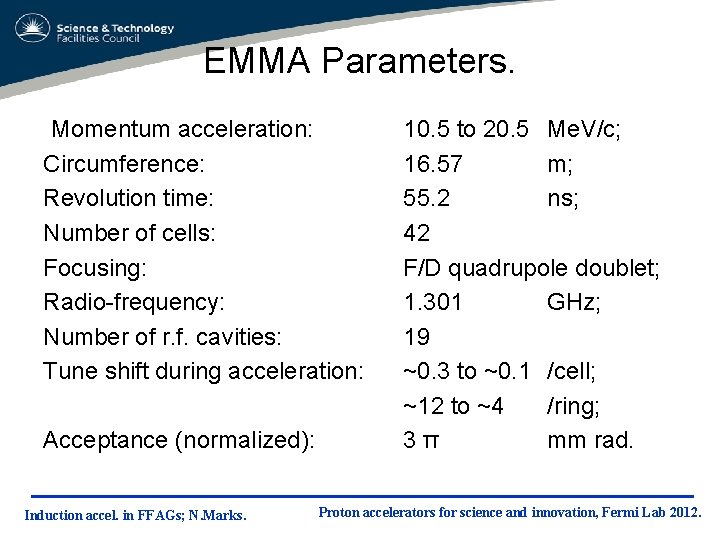
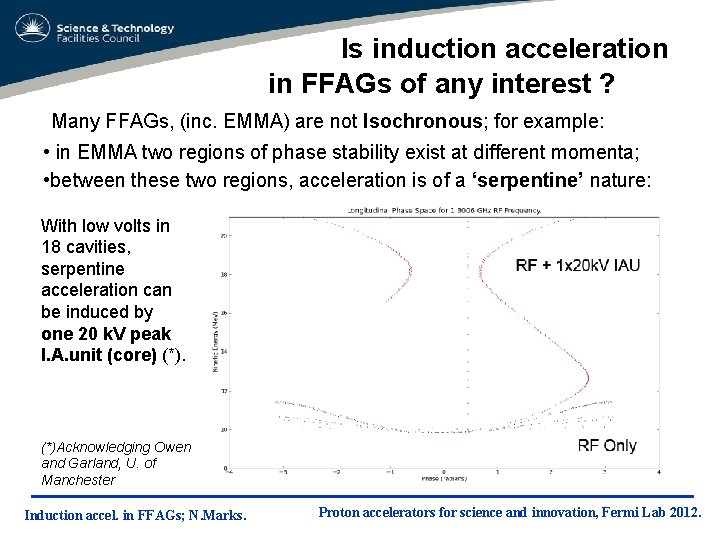
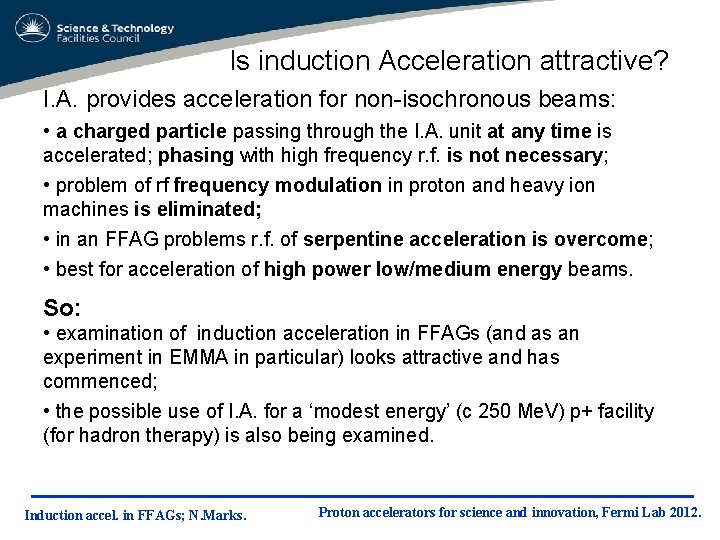
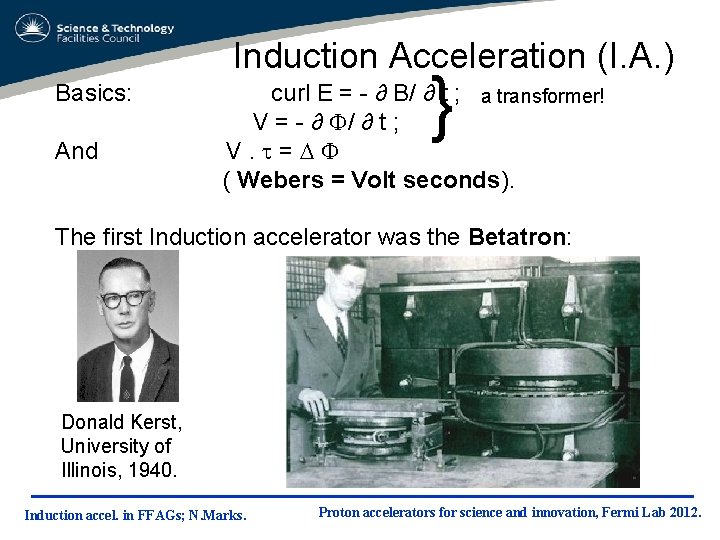
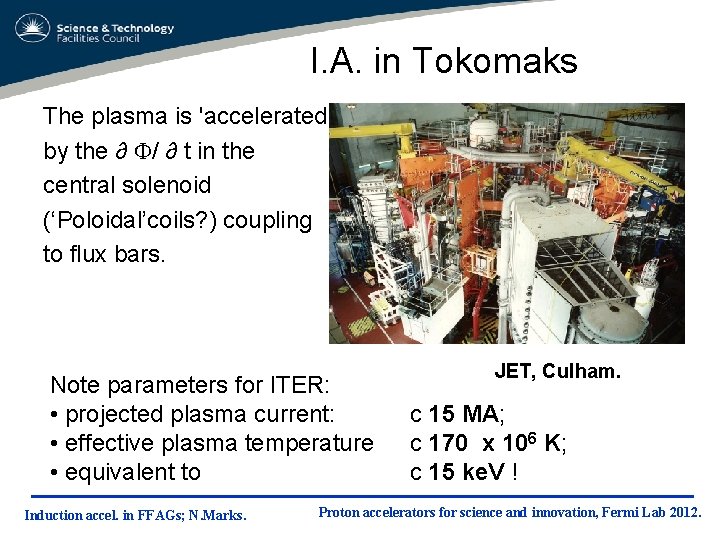
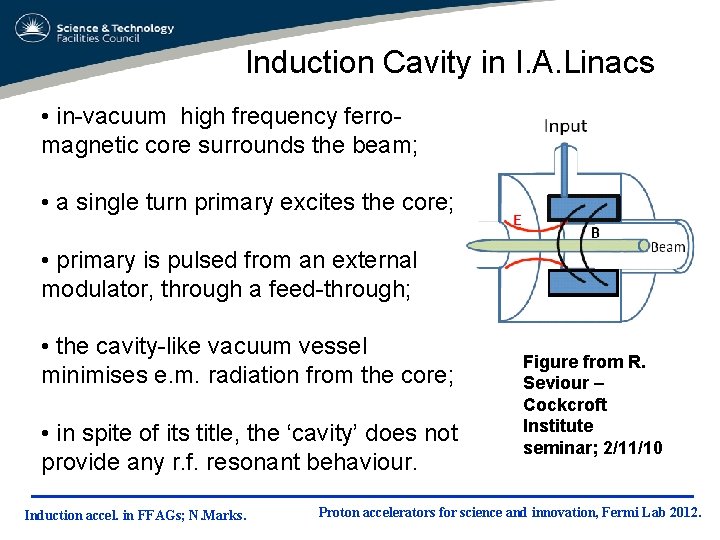
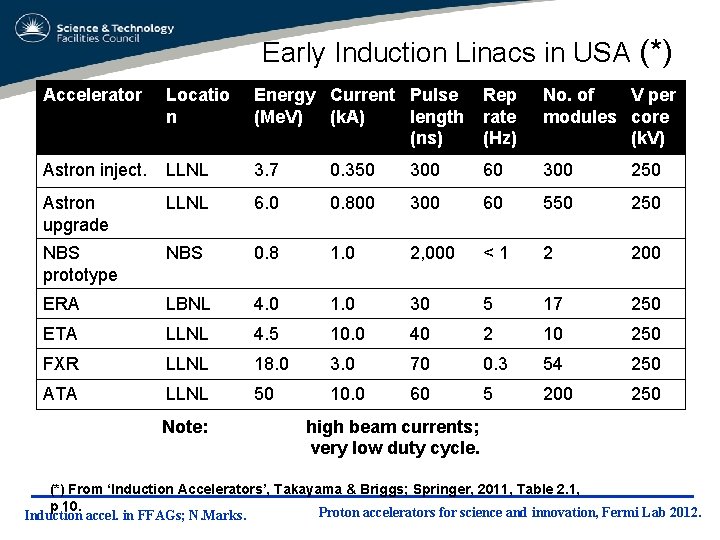
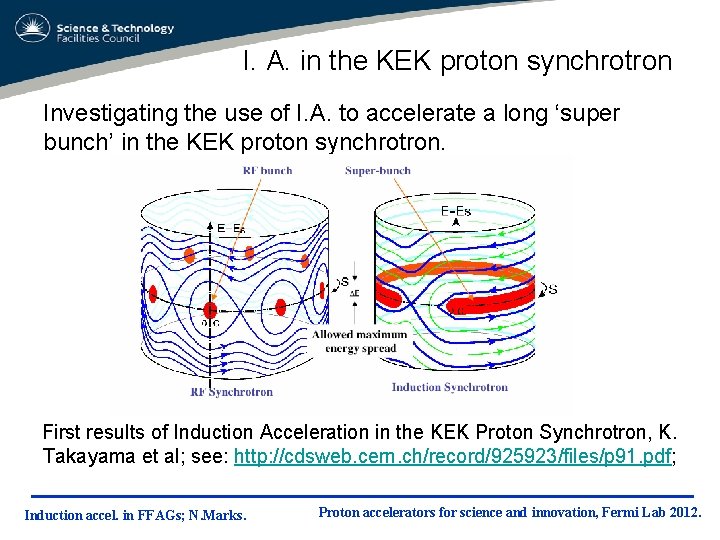
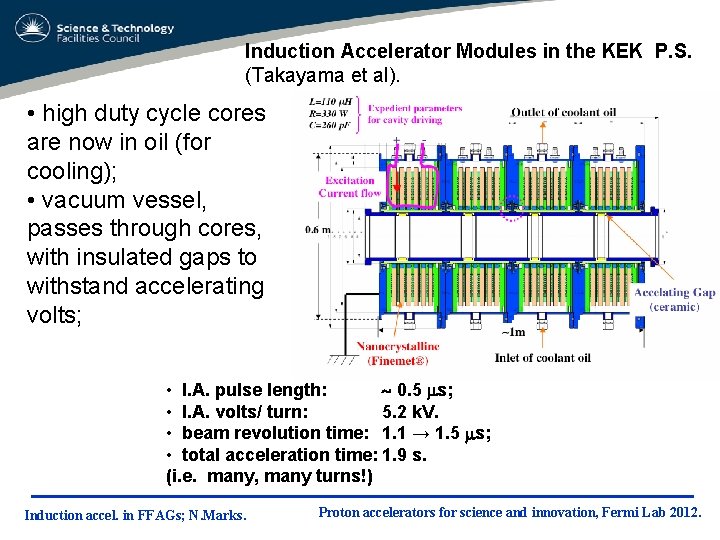
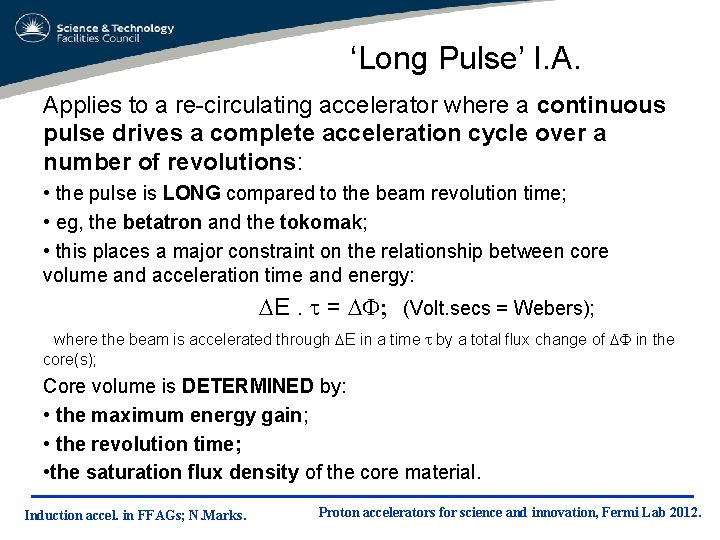
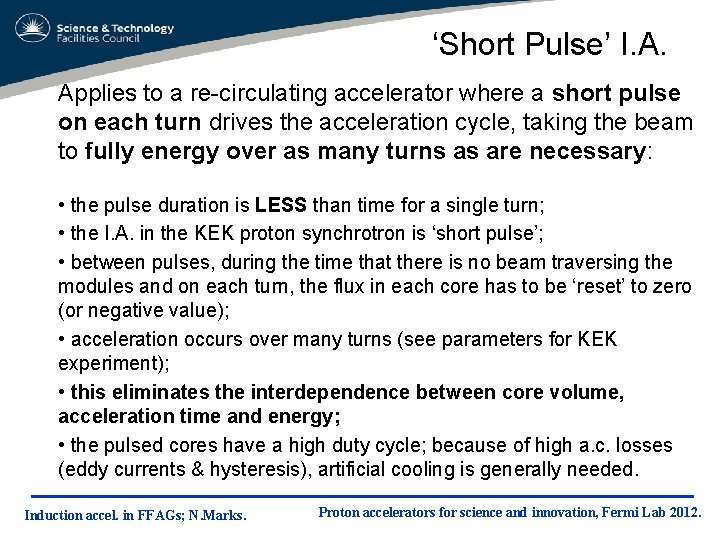
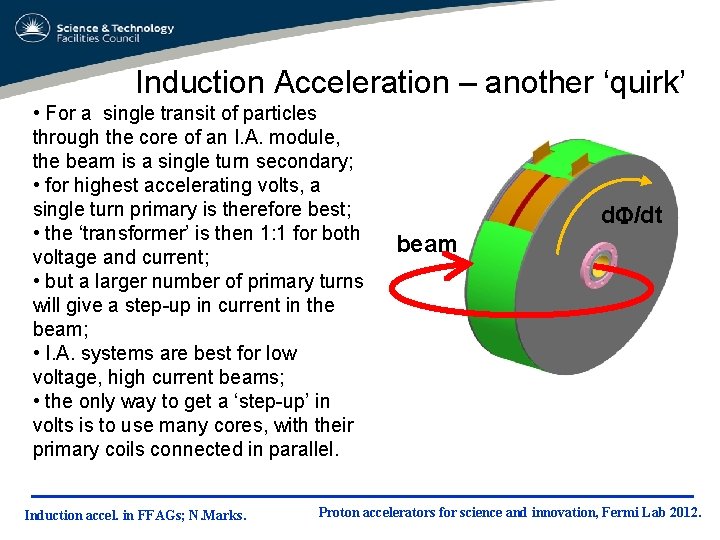
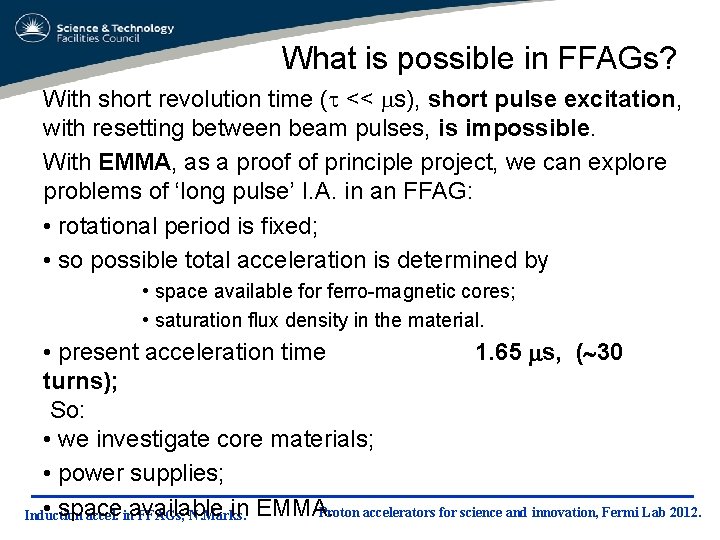
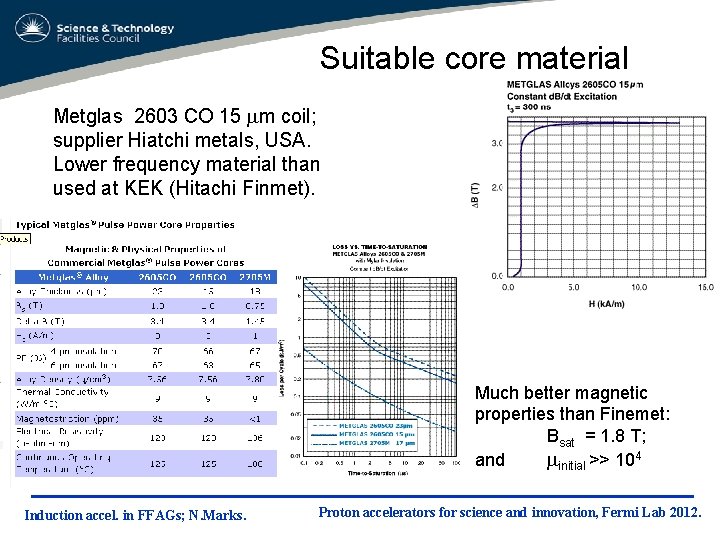
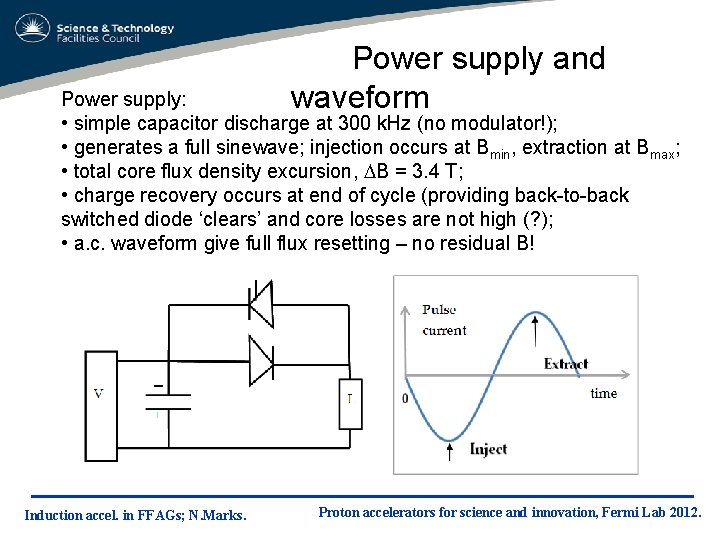
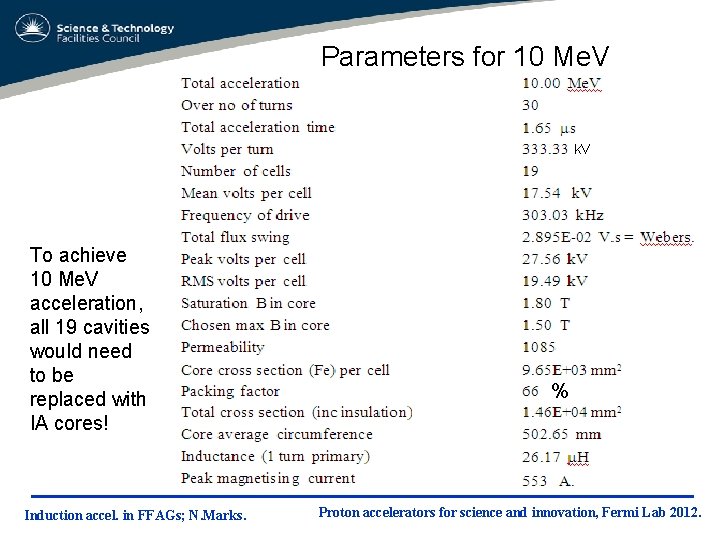
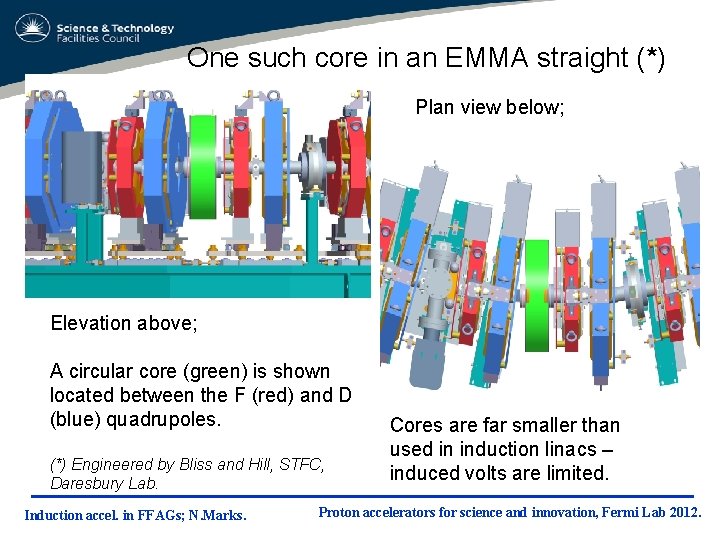
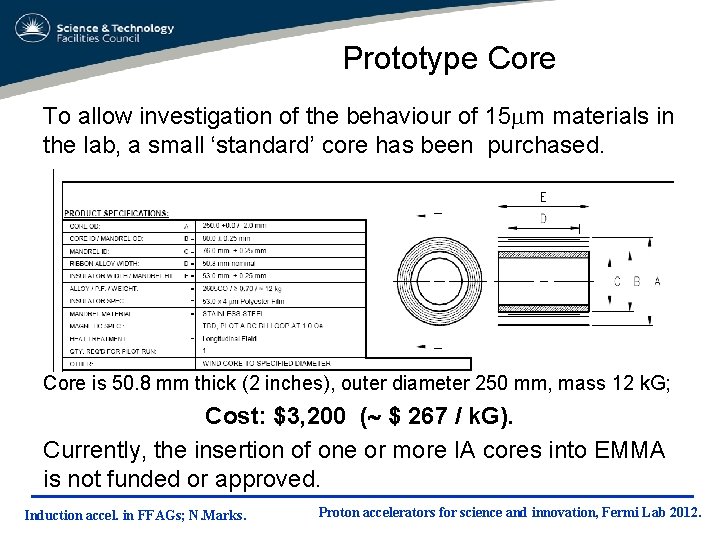
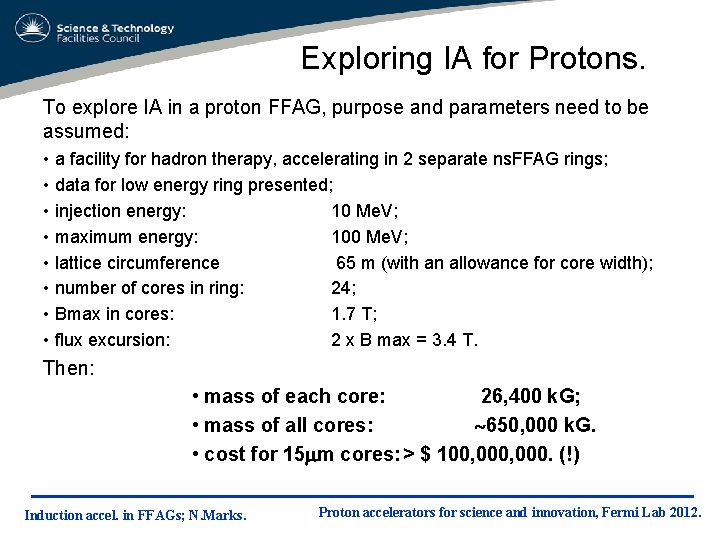
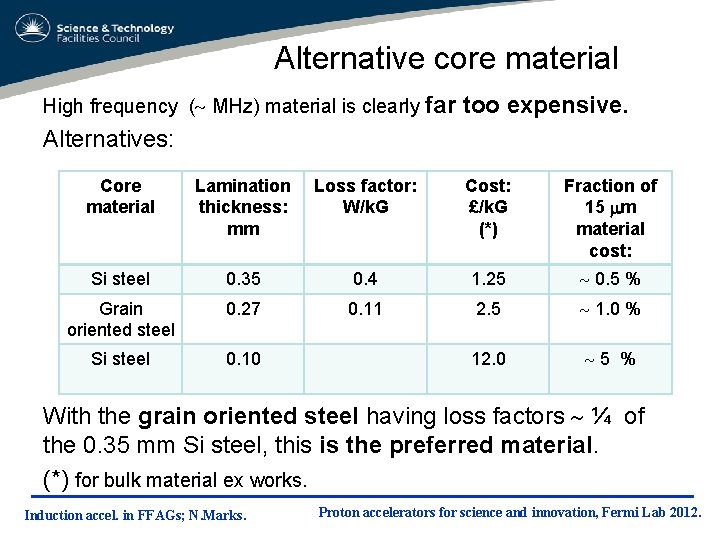
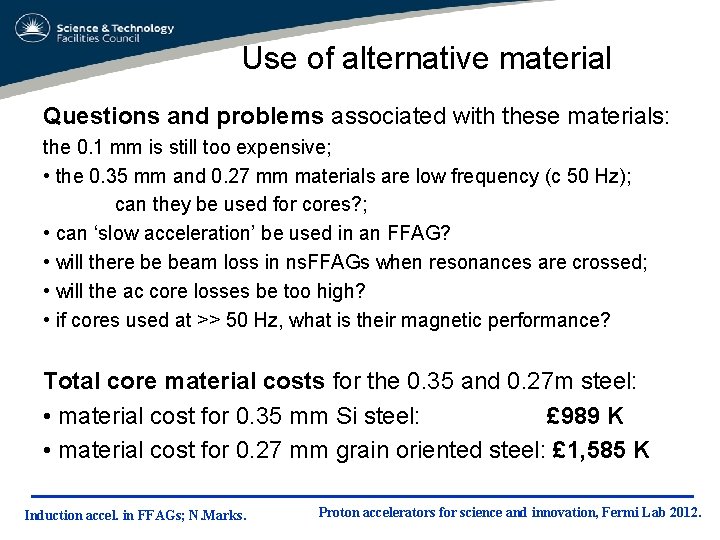
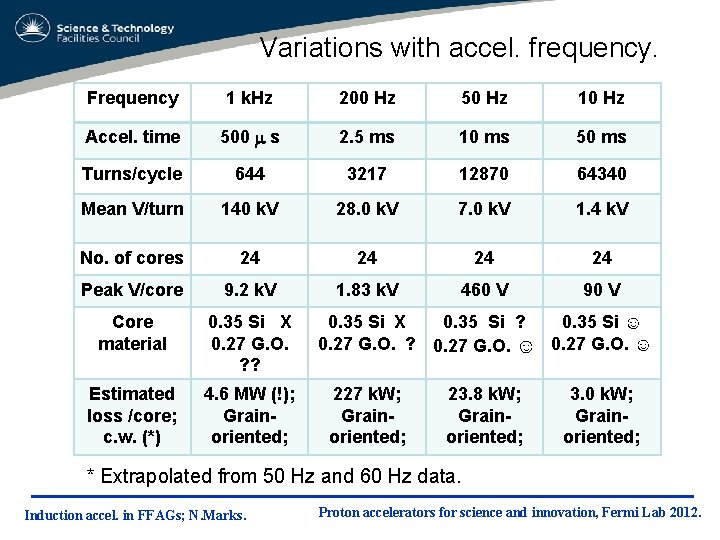
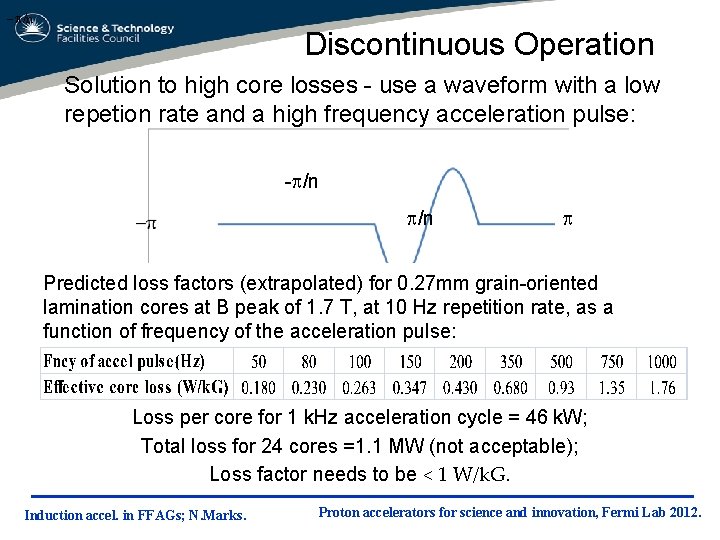
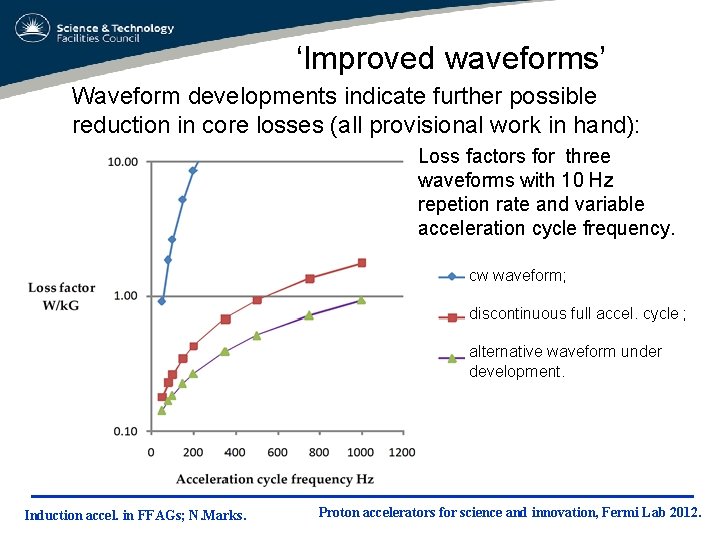
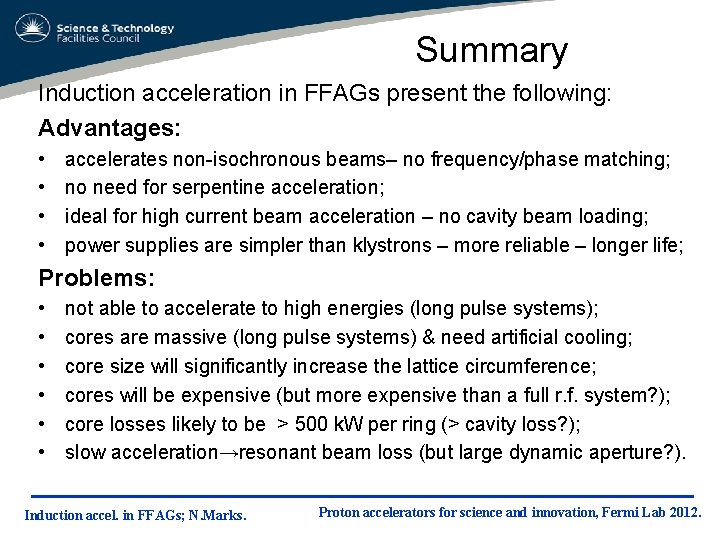
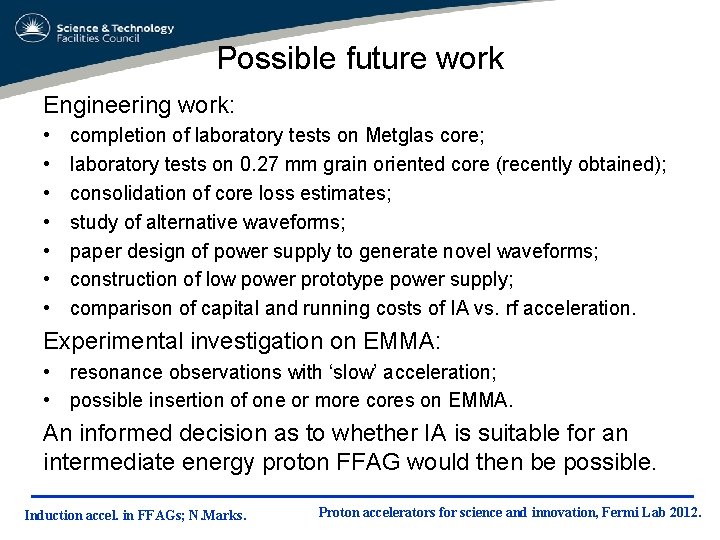
- Slides: 34
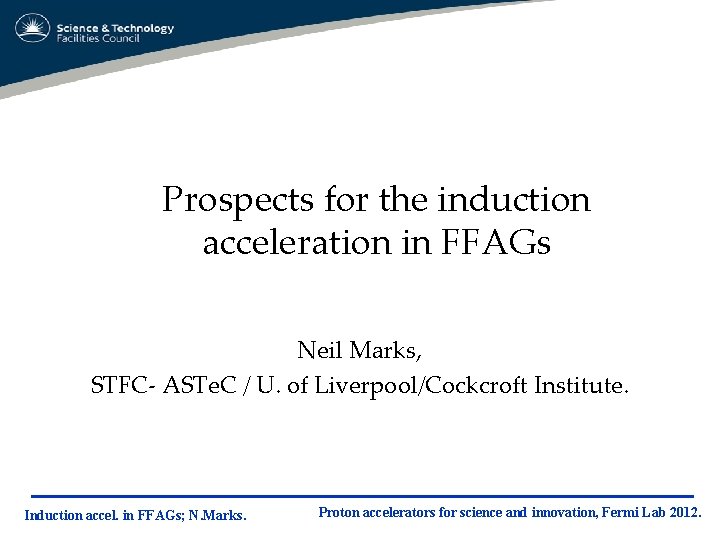
Prospects for the induction acceleration in FFAGs Neil Marks, STFC- ASTe. C / U. of Liverpool/Cockcroft Institute. Induction accel. in FFAGs; N. Marks. Proton accelerators for science and innovation, Fermi Lab 2012.
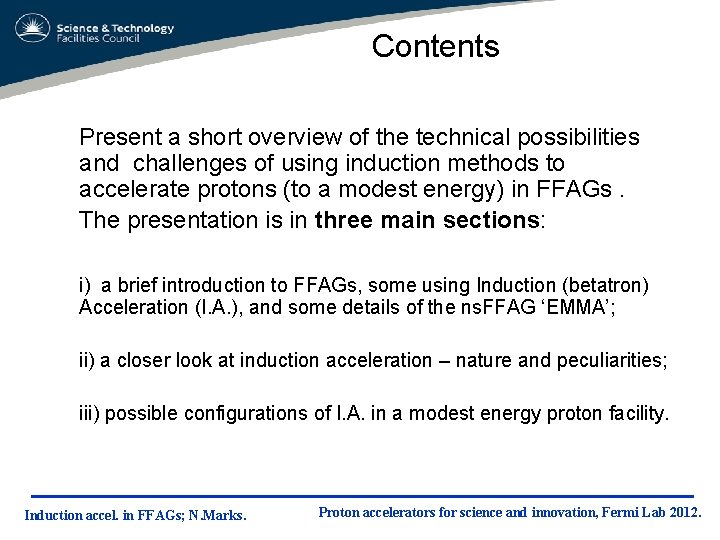
Contents Present a short overview of the technical possibilities and challenges of using induction methods to accelerate protons (to a modest energy) in FFAGs. The presentation is in three main sections: i) a brief introduction to FFAGs, some using Induction (betatron) Acceleration (I. A. ), and some details of the ns. FFAG ‘EMMA’; ii) a closer look at induction acceleration – nature and peculiarities; iii) possible configurations of I. A. in a modest energy proton facility. Induction accel. in FFAGs; N. Marks. Proton accelerators for science and innovation, Fermi Lab 2012.
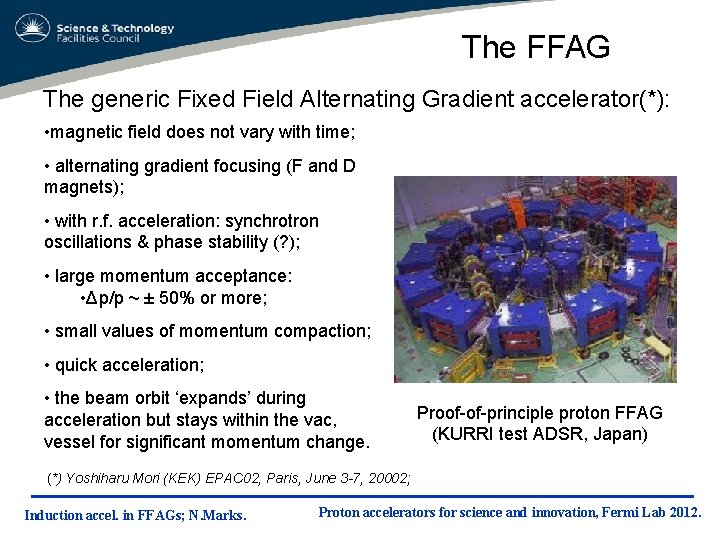
The FFAG The generic Fixed Field Alternating Gradient accelerator(*): • magnetic field does not vary with time; • alternating gradient focusing (F and D magnets); • with r. f. acceleration: synchrotron oscillations & phase stability (? ); eg: Proof-of-principle proton FFAG (KURRI test ADSR, Japan) • large momentum acceptance: • Δp/p ~ ± 50% or more; • small values of momentum compaction; • quick acceleration; • the beam orbit ‘expands’ during acceleration but stays within the vac, vessel for significant momentum change. Proof-of-principle proton FFAG (KURRI test ADSR, Japan) (*) Yoshiharu Mori (KEK) EPAC 02, Paris, June 3 -7, 20002; Induction accel. in FFAGs; N. Marks. Proton accelerators for science and innovation, Fermi Lab 2012.
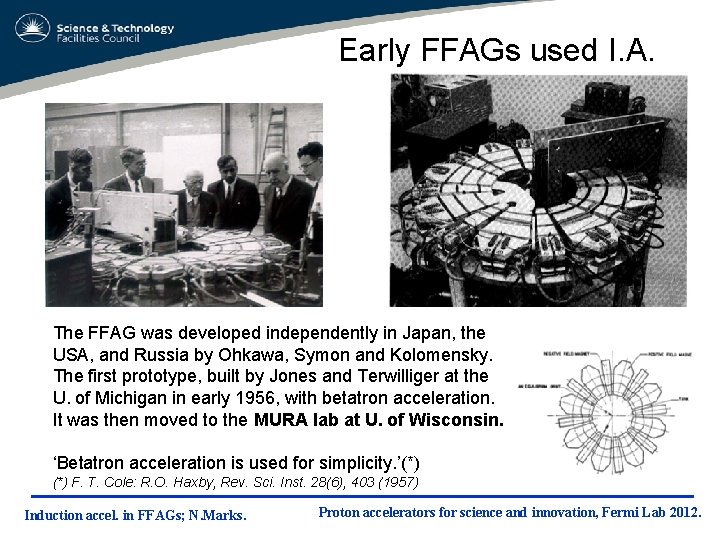
Early FFAGs used I. A. The FFAG was developed independently in Japan, the USA, and Russia by Ohkawa, Symon and Kolomensky. The first prototype, built by Jones and Terwilliger at the U. of Michigan in early 1956, with betatron acceleration. It was then moved to the MURA lab at U. of Wisconsin. ‘Betatron acceleration is used for simplicity. ’(*) F. T. Cole: R. O. Haxby, Rev. Sci. Inst. 28(6), 403 (1957) Induction accel. in FFAGs; N. Marks. Proton accelerators for science and innovation, Fermi Lab 2012.
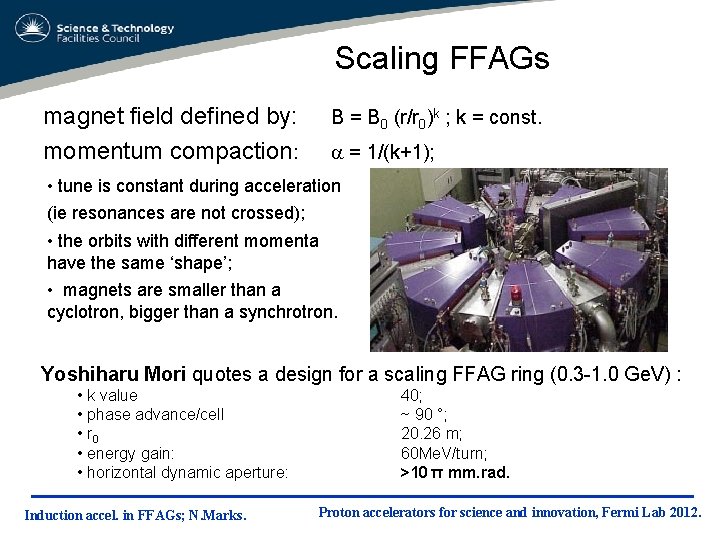
Scaling FFAGs magnet field defined by: momentum compaction: B = B 0 (r/r 0)k ; k = const. a = 1/(k+1); • tune is constant during acceleration (ie resonances are not crossed); • the orbits with different momenta have the same ‘shape’; • magnets are smaller than a cyclotron, bigger than a synchrotron. Yoshiharu Mori quotes a design for a scaling FFAG ring (0. 3 -1. 0 Ge. V) : • k value • phase advance/cell • r 0 • energy gain: • horizontal dynamic aperture: Induction accel. in FFAGs; N. Marks. 40; ~ 90 °; 20. 26 m; 60 Me. V/turn; >10 π mm. rad. Proton accelerators for science and innovation, Fermi Lab 2012.
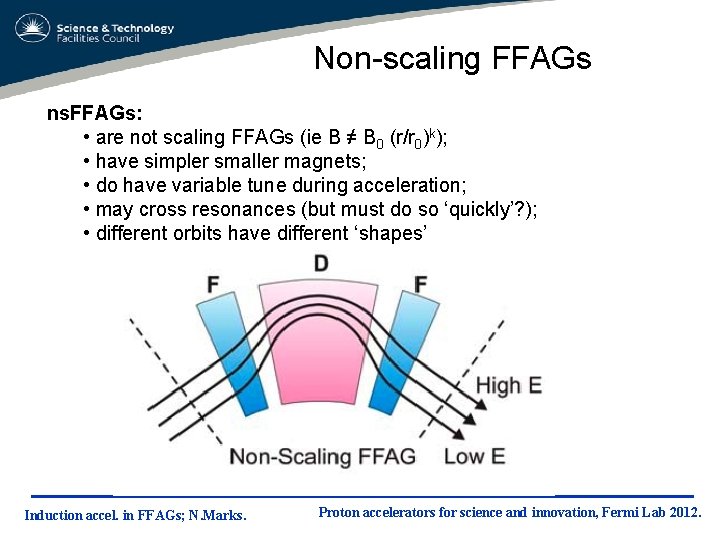
Non-scaling FFAGs ns. FFAGs: • are not scaling FFAGs (ie B ≠ B 0 (r/r 0)k); • have simpler smaller magnets; • do have variable tune during acceleration; • may cross resonances (but must do so ‘quickly’? ); • different orbits have different ‘shapes’ Induction accel. in FFAGs; N. Marks. Proton accelerators for science and innovation, Fermi Lab 2012.
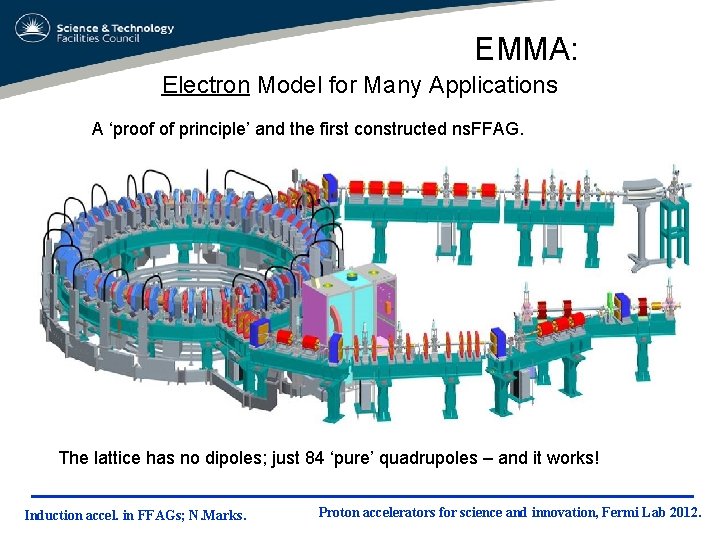
EMMA: Electron Model for Many Applications A ‘proof of principle’ and the first constructed ns. FFAG. The lattice has no dipoles; just 84 ‘pure’ quadrupoles – and it works! Induction accel. in FFAGs; N. Marks. Proton accelerators for science and innovation, Fermi Lab 2012.
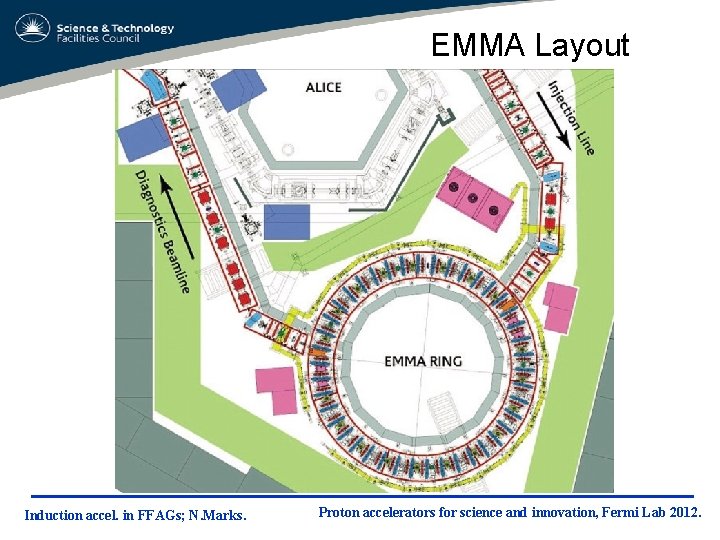
EMMA Layout Induction accel. in FFAGs; N. Marks. Proton accelerators for science and innovation, Fermi Lab 2012.
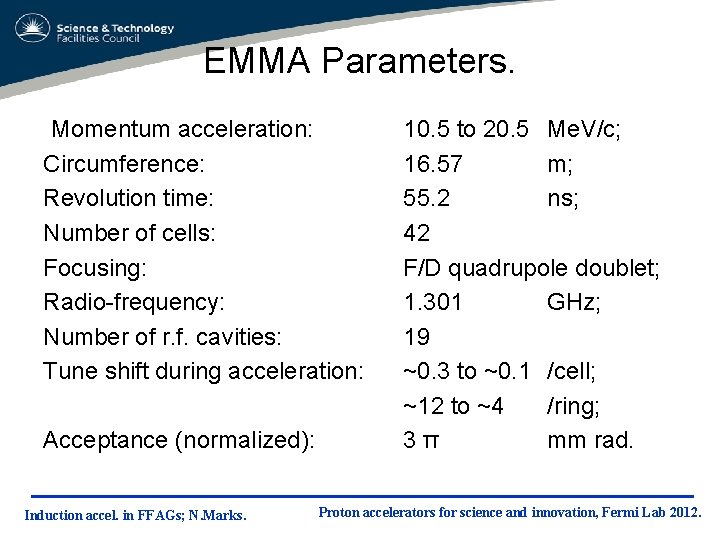
EMMA Parameters. Momentum acceleration: Circumference: Revolution time: Number of cells: Focusing: Radio-frequency: Number of r. f. cavities: Tune shift during acceleration: Acceptance (normalized): Induction accel. in FFAGs; N. Marks. 10. 5 to 20. 5 Me. V/c; 16. 57 m; 55. 2 ns; 42 F/D quadrupole doublet; 1. 301 GHz; 19 ~0. 3 to ~0. 1 /cell; ~12 to ~4 /ring; 3π mm rad. Proton accelerators for science and innovation, Fermi Lab 2012.
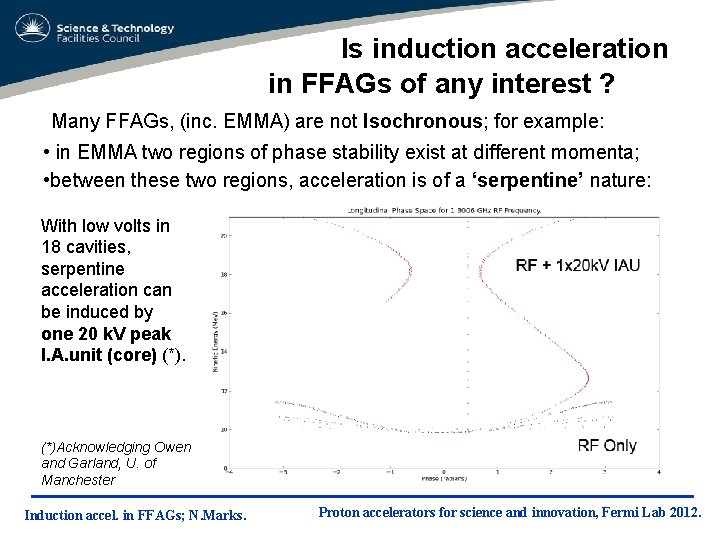
Is induction acceleration in FFAGs of any interest ? Many FFAGs, (inc. EMMA) are not Isochronous; for example: • in EMMA two regions of phase stability exist at different momenta; • between these two regions, acceleration is of a ‘serpentine’ nature: With low volts in 18 cavities, serpentine acceleration can be induced by one 20 k. V peak I. A. unit (core) (*)Acknowledging Owen and Garland, U. of Manchester Induction accel. in FFAGs; N. Marks. Proton accelerators for science and innovation, Fermi Lab 2012.
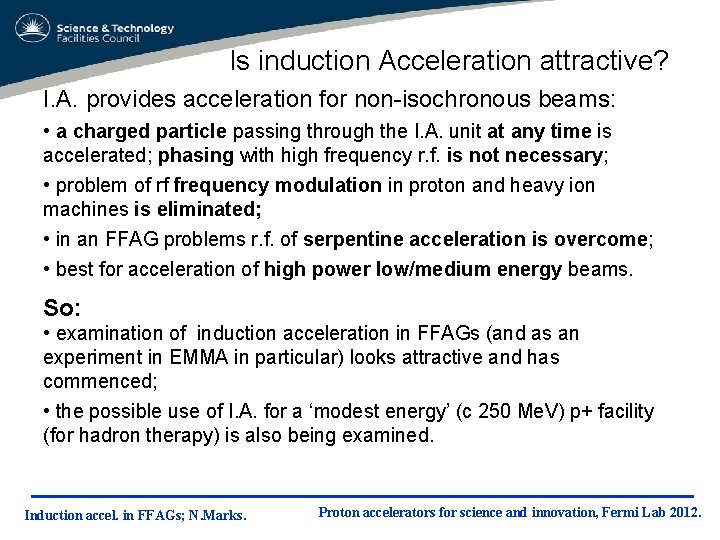
Is induction Acceleration attractive? I. A. provides acceleration for non-isochronous beams: • a charged particle passing through the I. A. unit at any time is accelerated; phasing with high frequency r. f. is not necessary; • problem of rf frequency modulation in proton and heavy ion machines is eliminated; • in an FFAG problems r. f. of serpentine acceleration is overcome; • best for acceleration of high power low/medium energy beams. So: • examination of induction acceleration in FFAGs (and as an experiment in EMMA in particular) looks attractive and has commenced; • the possible use of I. A. for a ‘modest energy’ (c 250 Me. V) p+ facility (for hadron therapy) is also being examined. Induction accel. in FFAGs; N. Marks. Proton accelerators for science and innovation, Fermi Lab 2012.
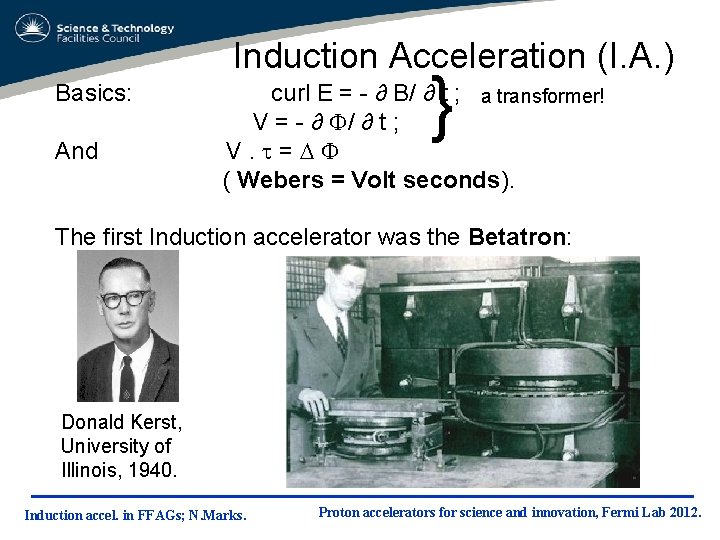
Induction Acceleration (I. A. ) Basics: And } curl E = - ∂ B/ ∂ t ; a transformer! V = - ∂ F/ ∂ t ; V. t=DF ( Webers = Volt seconds). The first Induction accelerator was the Betatron: Donald Kerst, University of Illinois, 1940. Induction accel. in FFAGs; N. Marks. Proton accelerators for science and innovation, Fermi Lab 2012.
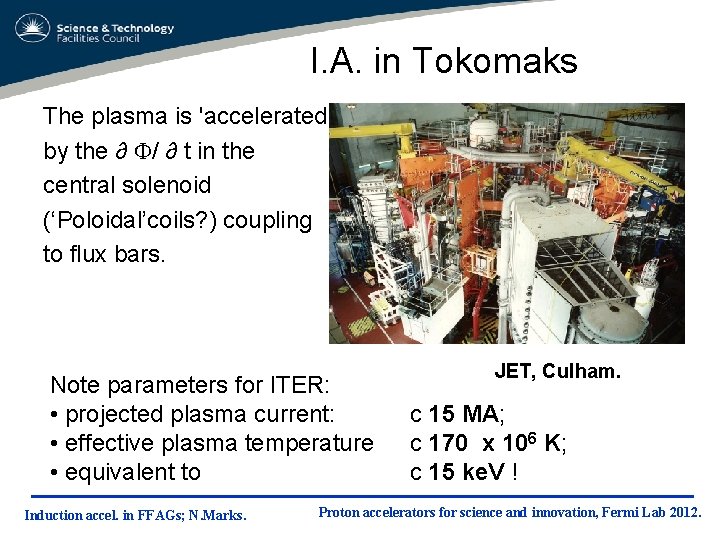
I. A. in Tokomaks The plasma is 'accelerated’ by the ∂ F/ ∂ t in the central solenoid (‘Poloidal’coils? ) coupling to flux bars. Note parameters for ITER: • projected plasma current: • effective plasma temperature • equivalent to Induction accel. in FFAGs; N. Marks. JET, Culham. c 15 MA; c 170 x 106 K; c 15 ke. V ! Proton accelerators for science and innovation, Fermi Lab 2012.
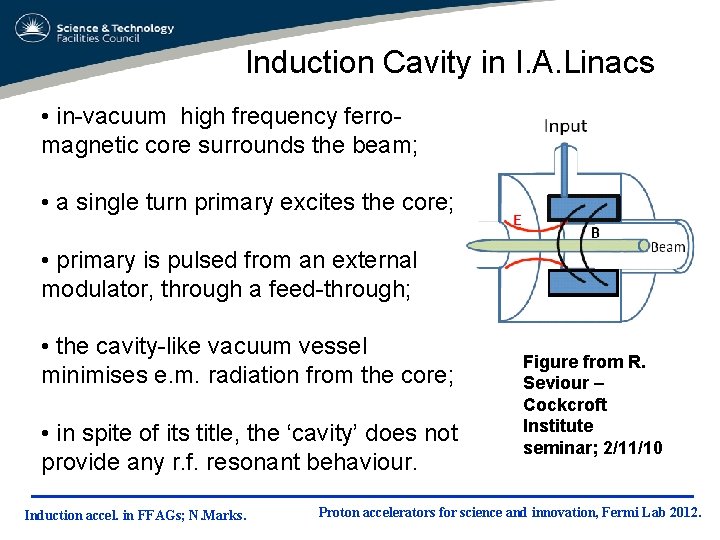
Induction Cavity in I. A. Linacs • in-vacuum high frequency ferromagnetic core surrounds the beam; • a single turn primary excites the core; • primary is pulsed from an external modulator, through a feed-through; • the cavity-like vacuum vessel minimises e. m. radiation from the core; • in spite of its title, the ‘cavity’ does not provide any r. f. resonant behaviour. Induction accel. in FFAGs; N. Marks. Figure from R. Seviour – Cockcroft Institute seminar; 2/11/10 Proton accelerators for science and innovation, Fermi Lab 2012.
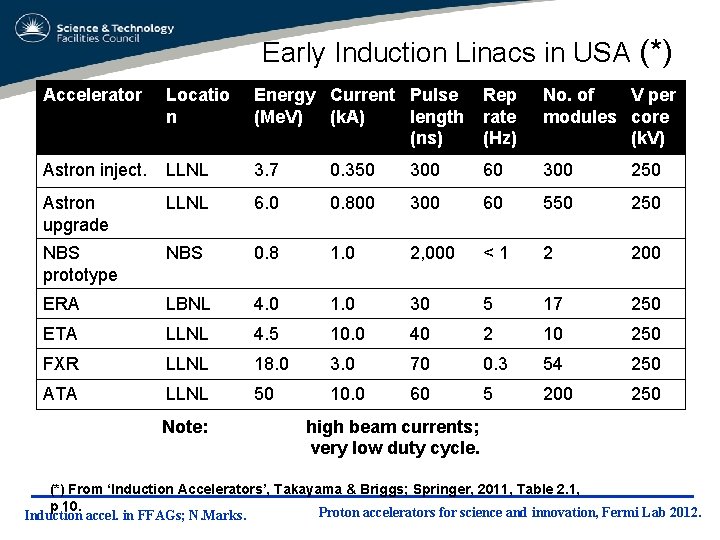
Early Induction Linacs in USA (*) Accelerator Locatio n Energy Current Pulse (Me. V) (k. A) length (ns) Rep rate (Hz) No. of V per modules core (k. V) Astron inject. LLNL 3. 7 0. 350 300 60 300 250 Astron upgrade LLNL 6. 0 0. 800 300 60 550 250 NBS prototype NBS 0. 8 1. 0 2, 000 <1 2 200 ERA LBNL 4. 0 1. 0 30 5 17 250 ETA LLNL 4. 5 10. 0 40 2 10 250 FXR LLNL 18. 0 3. 0 70 0. 3 54 250 ATA LLNL 50 10. 0 60 5 200 250 Note: high beam currents; very low duty cycle. (*) From ‘Induction Accelerators’, Takayama & Briggs; Springer, 2011, Table 2. 1, p 10. Proton accelerators for science and innovation, Fermi Lab 2012. Induction accel. in FFAGs; N. Marks.
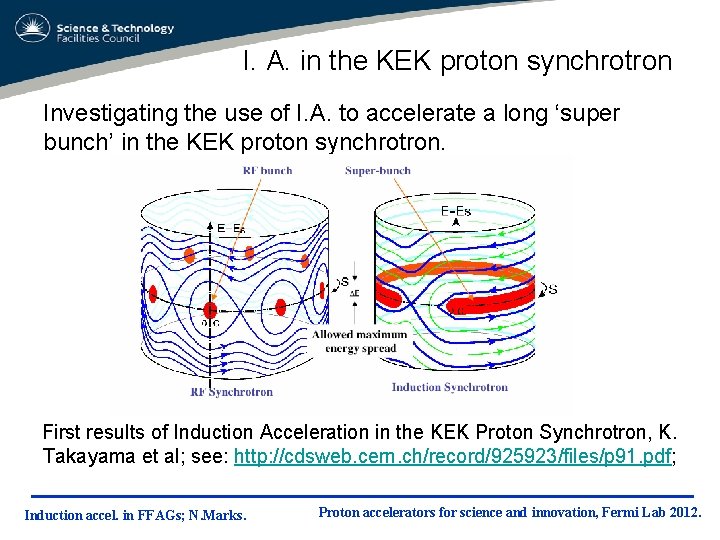
I. A. in the KEK proton synchrotron Investigating the use of I. A. to accelerate a long ‘super bunch’ in the KEK proton synchrotron. First results of Induction Acceleration in the KEK Proton Synchrotron, K. Takayama et al; see: http: //cdsweb. cern. ch/record/925923/files/p 91. pdf; Induction accel. in FFAGs; N. Marks. Proton accelerators for science and innovation, Fermi Lab 2012.
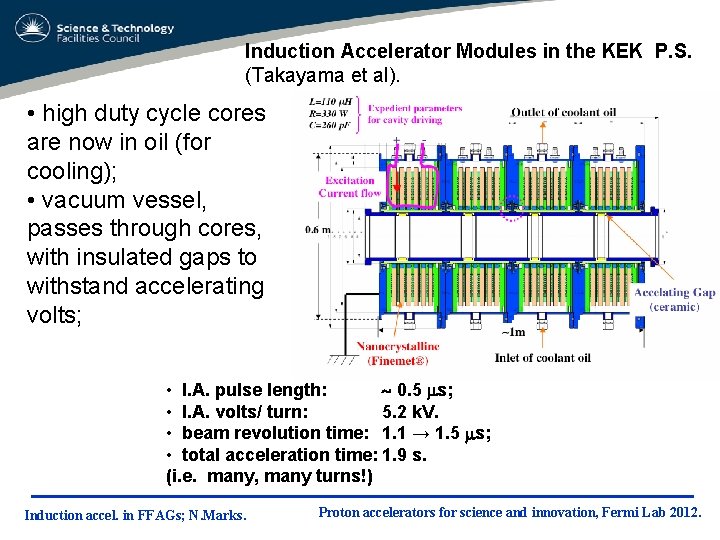
Induction Accelerator Modules in the KEK P. S. (Takayama et al). • high duty cycle cores are now in oil (for cooling); • vacuum vessel, passes through cores, with insulated gaps to withstand accelerating volts; • I. A. pulse length: 0. 5 ms; • I. A. volts/ turn: 5. 2 k. V. • beam revolution time: 1. 1 → 1. 5 ms; • total acceleration time: 1. 9 s. (i. e. many, many turns!) Induction accel. in FFAGs; N. Marks. Proton accelerators for science and innovation, Fermi Lab 2012.
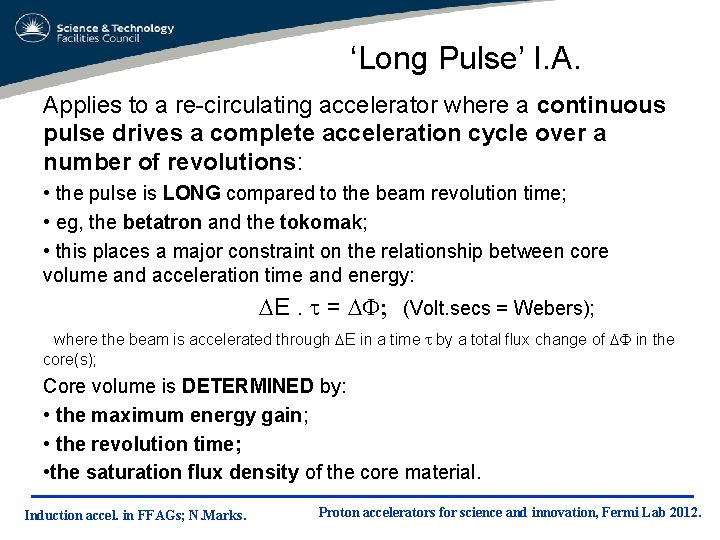
‘Long Pulse’ I. A. Applies to a re-circulating accelerator where a continuous pulse drives a complete acceleration cycle over a number of revolutions: • the pulse is LONG compared to the beam revolution time; • eg, the betatron and the tokomak; • this places a major constraint on the relationship between core volume and acceleration time and energy: DE. t = DF; (Volt. secs = Webers); where the beam is accelerated through DE in a time t by a total flux change of DF in the core(s); Core volume is DETERMINED by: • the maximum energy gain; • the revolution time; • the saturation flux density of the core material. Induction accel. in FFAGs; N. Marks. Proton accelerators for science and innovation, Fermi Lab 2012.
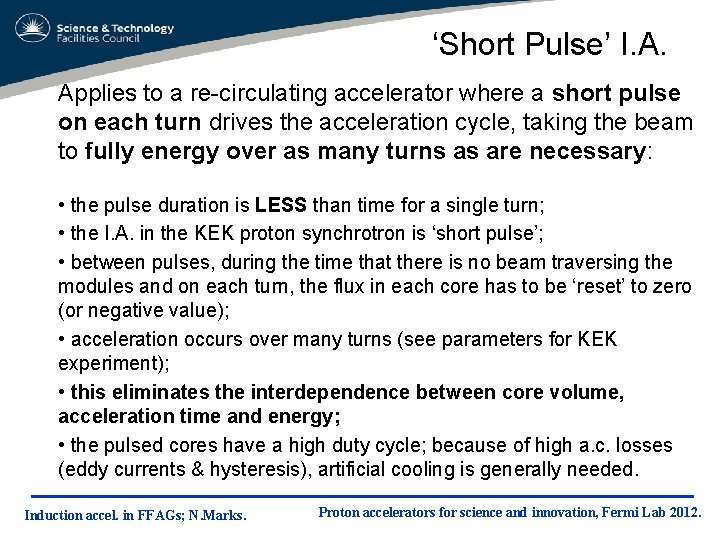
‘Short Pulse’ I. A. Applies to a re-circulating accelerator where a short pulse on each turn drives the acceleration cycle, taking the beam to fully energy over as many turns as are necessary: • the pulse duration is LESS than time for a single turn; • the I. A. in the KEK proton synchrotron is ‘short pulse’; • between pulses, during the time that there is no beam traversing the modules and on each turn, the flux in each core has to be ‘reset’ to zero (or negative value); • acceleration occurs over many turns (see parameters for KEK experiment); • this eliminates the interdependence between core volume, acceleration time and energy; • the pulsed cores have a high duty cycle; because of high a. c. losses (eddy currents & hysteresis), artificial cooling is generally needed. Induction accel. in FFAGs; N. Marks. Proton accelerators for science and innovation, Fermi Lab 2012.
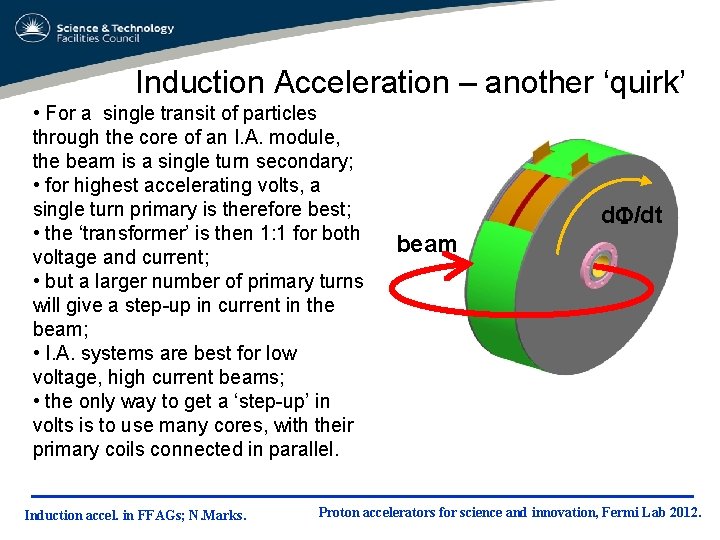
Induction Acceleration – another ‘quirk’ • For a single transit of particles through the core of an I. A. module, the beam is a single turn secondary; • for highest accelerating volts, a single turn primary is therefore best; • the ‘transformer’ is then 1: 1 for both voltage and current; • but a larger number of primary turns will give a step-up in current in the beam; • I. A. systems are best for low voltage, high current beams; • the only way to get a ‘step-up’ in volts is to use many cores, with their primary coils connected in parallel. Induction accel. in FFAGs; N. Marks. d. F/dt beam Proton accelerators for science and innovation, Fermi Lab 2012.
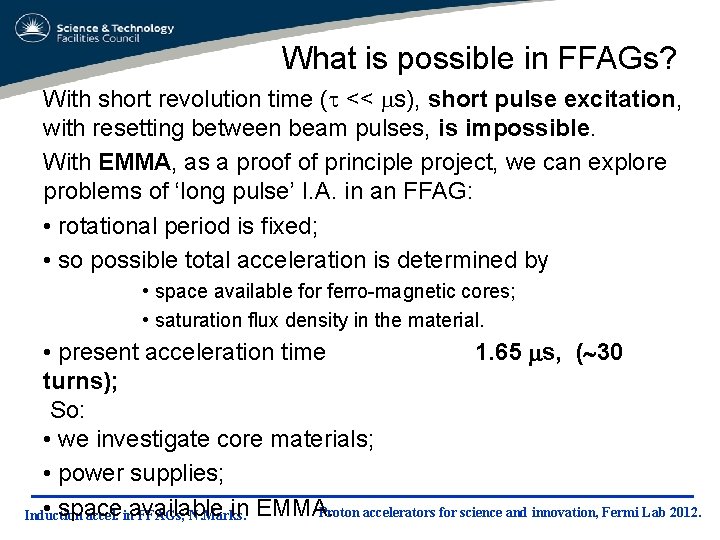
What is possible in FFAGs? With short revolution time (t << ms), short pulse excitation, with resetting between beam pulses, is impossible. With EMMA, as a proof of principle project, we can explore problems of ‘long pulse’ I. A. in an FFAG: • rotational period is fixed; • so possible total acceleration is determined by • space available for ferro-magnetic cores; • saturation flux density in the material. • present acceleration time 1. 65 ms, ( 30 turns); So: • we investigate core materials; • power supplies; • space in EMMA. Proton accelerators for science and innovation, Fermi Lab 2012. Induction accel. inavailable FFAGs; N. Marks.
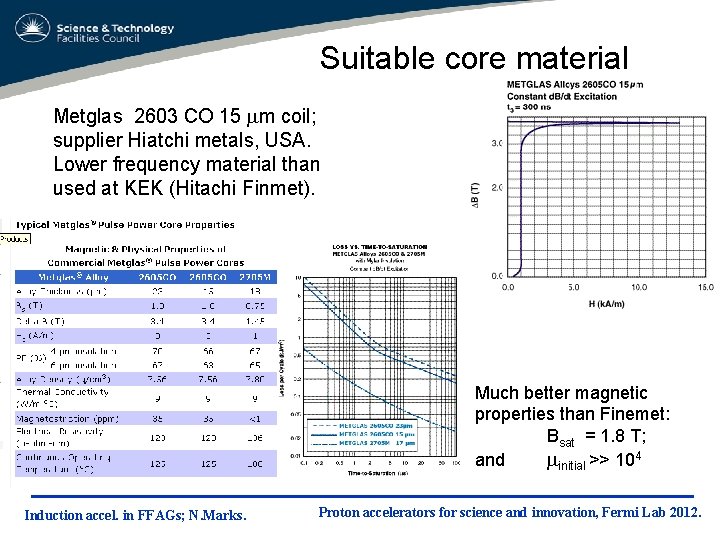
Suitable core material Metglas 2603 CO 15 mm coil; supplier Hiatchi metals, USA. Lower frequency material than used at KEK (Hitachi Finmet). Much better magnetic properties than Finemet: Bsat = 1. 8 T; and minitial >> 104 Induction accel. in FFAGs; N. Marks. Proton accelerators for science and innovation, Fermi Lab 2012.
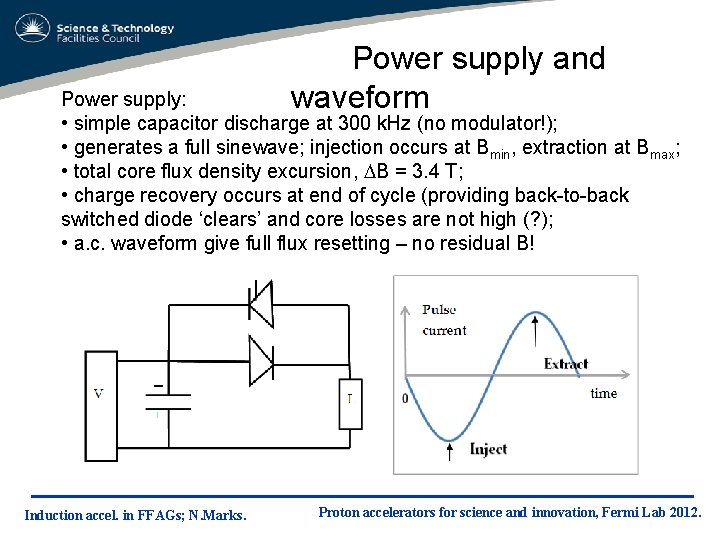
Power supply and waveform Power supply: • simple capacitor discharge at 300 k. Hz (no modulator!); • generates a full sinewave; injection occurs at Bmin, extraction at Bmax; • total core flux density excursion, DB = 3. 4 T; • charge recovery occurs at end of cycle (providing back-to-back switched diode ‘clears’ and core losses are not high (? ); • a. c. waveform give full flux resetting – no residual B! Induction accel. in FFAGs; N. Marks. Proton accelerators for science and innovation, Fermi Lab 2012.
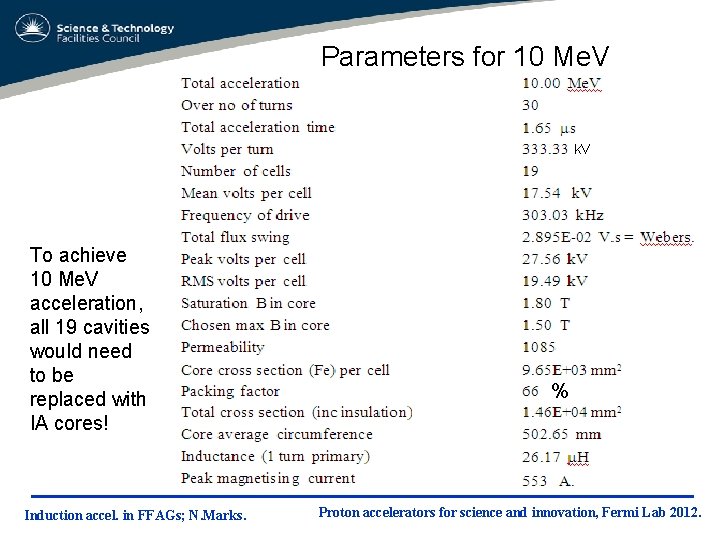
Parameters for 10 Me. V acceleration. k. V To achieve 10 Me. V acceleration, all 19 cavities would need to be replaced with IA cores! Induction accel. in FFAGs; N. Marks. % Proton accelerators for science and innovation, Fermi Lab 2012.
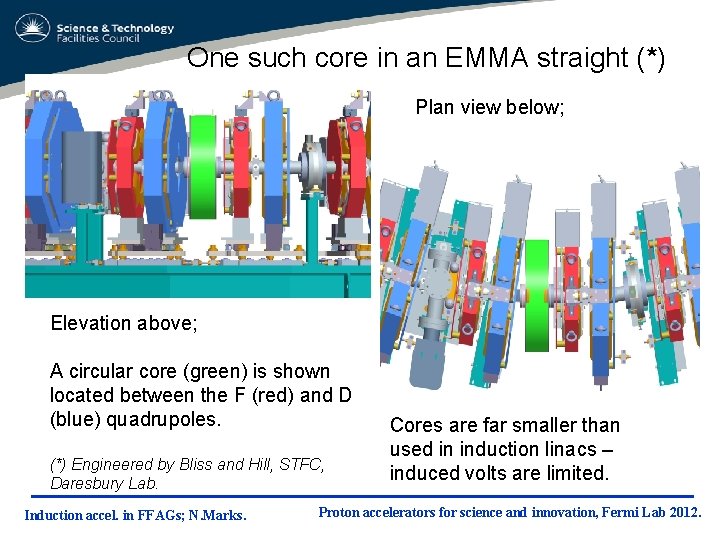
One such core in an EMMA straight (*) Plan view below; Elevation above; A circular core (green) is shown located between the F (red) and D (blue) quadrupoles. (*) Engineered by Bliss and Hill, STFC, Daresbury Lab. Induction accel. in FFAGs; N. Marks. Cores are far smaller than used in induction linacs – induced volts are limited. Proton accelerators for science and innovation, Fermi Lab 2012.
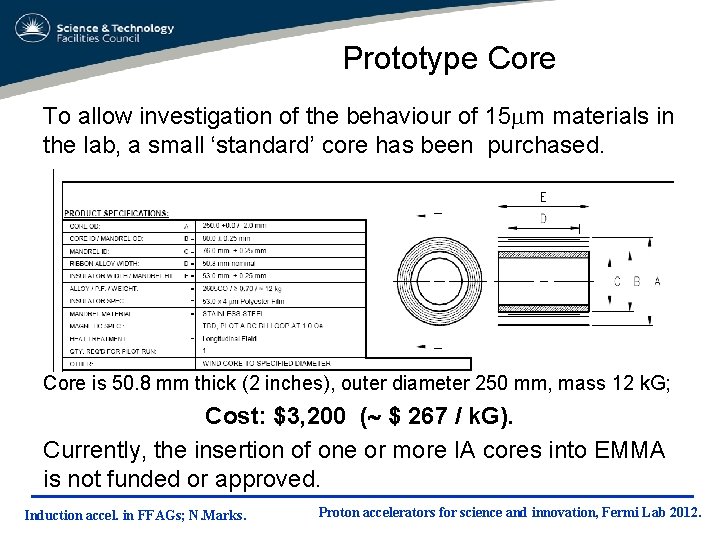
Prototype Core To allow investigation of the behaviour of 15 mm materials in the lab, a small ‘standard’ core has been purchased. Core is 50. 8 mm thick (2 inches), outer diameter 250 mm, mass 12 k. G; Cost: $3, 200 ( $ 267 / k. G). Currently, the insertion of one or more IA cores into EMMA is not funded or approved. Induction accel. in FFAGs; N. Marks. Proton accelerators for science and innovation, Fermi Lab 2012.
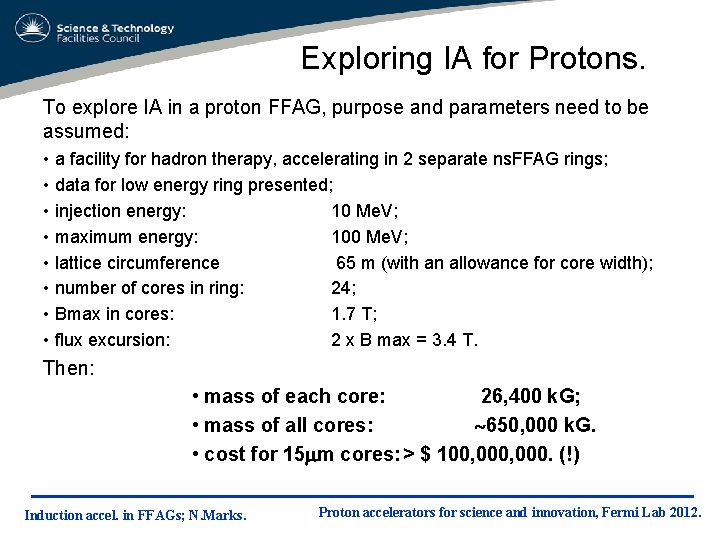
Exploring IA for Protons. To explore IA in a proton FFAG, purpose and parameters need to be assumed: • a facility for hadron therapy, accelerating in 2 separate ns. FFAG rings; • data for low energy ring presented; • injection energy: 10 Me. V; • maximum energy: 100 Me. V; • lattice circumference 65 m (with an allowance for core width); • number of cores in ring: 24; • Bmax in cores: 1. 7 T; • flux excursion: 2 x B max = 3. 4 T. Then: • mass of each core: 26, 400 k. G; • mass of all cores: 650, 000 k. G. • cost for 15 mm cores: > $ 100, 000. (!) Induction accel. in FFAGs; N. Marks. Proton accelerators for science and innovation, Fermi Lab 2012.
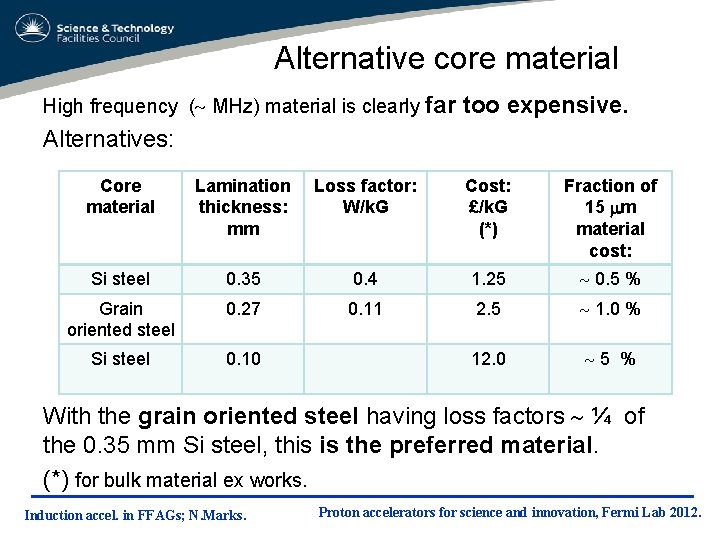
Alternative core material High frequency ( MHz) material is clearly far too expensive. Alternatives: Core material Lamination thickness: mm Loss factor: W/k. G Cost: £/k. G (*) Fraction of 15 mm material cost: Si steel 0. 35 0. 4 1. 25 0. 5 % Grain oriented steel 0. 27 0. 11 2. 5 1. 0 % Si steel 0. 10 12. 0 5 % With the grain oriented steel having loss factors ¼ of the 0. 35 mm Si steel, this is the preferred material. (*) for bulk material ex works. Induction accel. in FFAGs; N. Marks. Proton accelerators for science and innovation, Fermi Lab 2012.
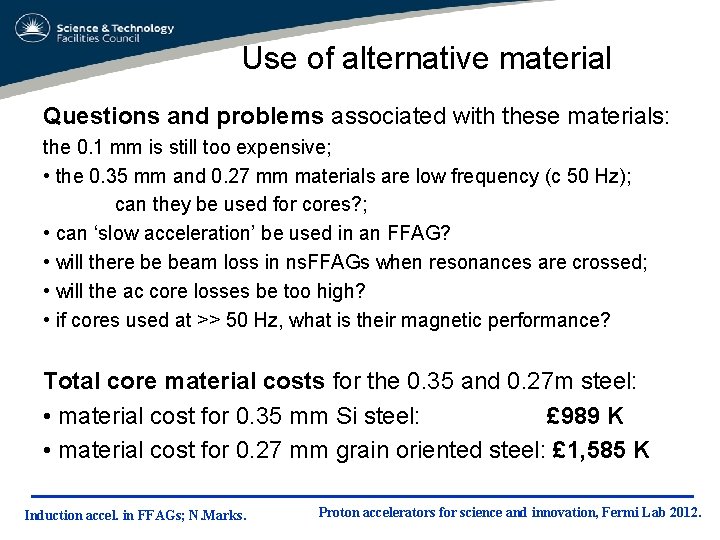
Use of alternative material Questions and problems associated with these materials: the 0. 1 mm is still too expensive; • the 0. 35 mm and 0. 27 mm materials are low frequency (c 50 Hz); can they be used for cores? ; • can ‘slow acceleration’ be used in an FFAG? • will there be beam loss in ns. FFAGs when resonances are crossed; • will the ac core losses be too high? • if cores used at >> 50 Hz, what is their magnetic performance? Total core material costs for the 0. 35 and 0. 27 m steel: • material cost for 0. 35 mm Si steel: £ 989 K • material cost for 0. 27 mm grain oriented steel: £ 1, 585 K Induction accel. in FFAGs; N. Marks. Proton accelerators for science and innovation, Fermi Lab 2012.
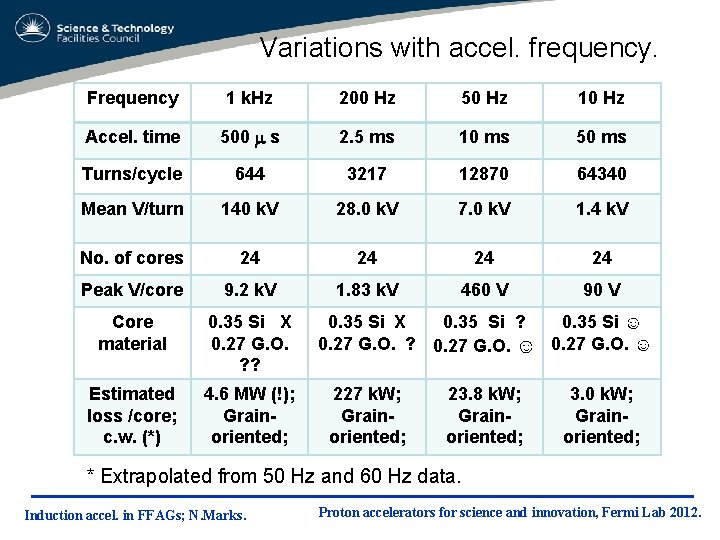
Variations with accel. frequency. Frequency 1 k. Hz 200 Hz 50 Hz 10 Hz Accel. time 500 m s 2. 5 ms 10 ms 50 ms Turns/cycle 644 3217 12870 64340 Mean V/turn 140 k. V 28. 0 k. V 7. 0 k. V 1. 4 k. V No. of cores 24 24 Peak V/core 9. 2 k. V 1. 83 k. V 460 V 90 V Core material 0. 35 Si X 0. 27 G. O. ? ? Estimated loss /core; c. w. (*) 4. 6 MW (!); Grainoriented; 0. 35 Si X 0. 35 Si ? 0. 35 Si ☺ 0. 27 G. O. ? 0. 27 G. O. ☺ 227 k. W; Grainoriented; 23. 8 k. W; Grainoriented; 3. 0 k. W; Grainoriented; * Extrapolated from 50 Hz and 60 Hz data. Induction accel. in FFAGs; N. Marks. Proton accelerators for science and innovation, Fermi Lab 2012.
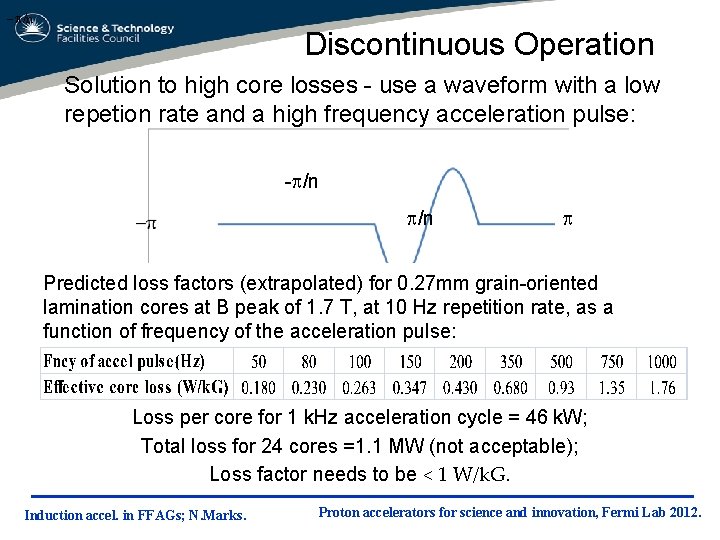
-p/n Discontinuous Operation Solution to high core losses - use a waveform with a low repetion rate and a high frequency acceleration pulse: -p/n p Predicted loss factors (extrapolated) for 0. 27 mm grain-oriented lamination cores at B peak of 1. 7 T, at 10 Hz repetition rate, as a function of frequency of the acceleration pulse: Loss per core for 1 k. Hz acceleration cycle = 46 k. W; Total loss for 24 cores =1. 1 MW (not acceptable); Loss factor needs to be < 1 W/k. G. Induction accel. in FFAGs; N. Marks. Proton accelerators for science and innovation, Fermi Lab 2012.
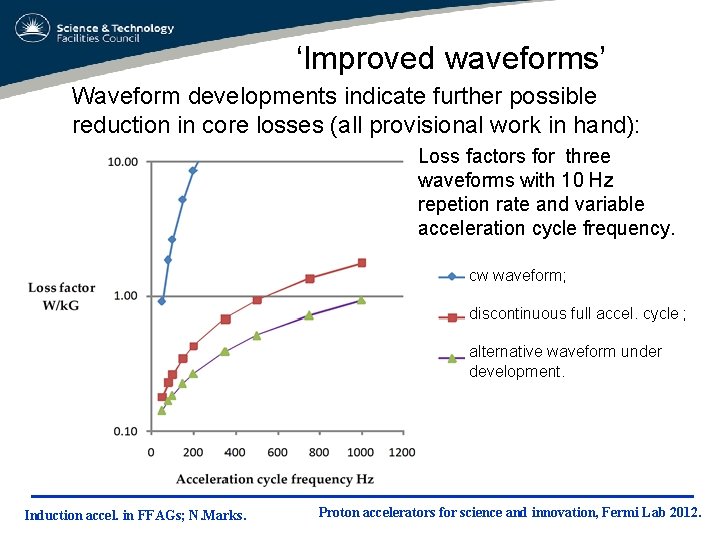
‘Improved waveforms’ Waveform developments indicate further possible reduction in core losses (all provisional work in hand): Loss factors for three waveforms with 10 Hz repetion rate and variable acceleration cycle frequency. cw waveform; discontinuous full accel. cycle ; alternative waveform under development. Induction accel. in FFAGs; N. Marks. Proton accelerators for science and innovation, Fermi Lab 2012.
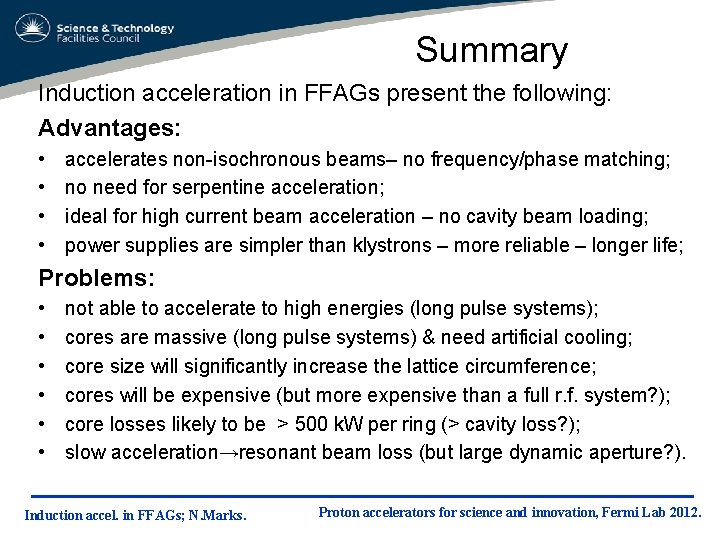
Summary Induction acceleration in FFAGs present the following: Advantages: • • accelerates non-isochronous beams– no frequency/phase matching; no need for serpentine acceleration; ideal for high current beam acceleration – no cavity beam loading; power supplies are simpler than klystrons – more reliable – longer life; Problems: • • • not able to accelerate to high energies (long pulse systems); cores are massive (long pulse systems) & need artificial cooling; core size will significantly increase the lattice circumference; cores will be expensive (but more expensive than a full r. f. system? ); core losses likely to be > 500 k. W per ring (> cavity loss? ); slow acceleration→resonant beam loss (but large dynamic aperture? ). Induction accel. in FFAGs; N. Marks. Proton accelerators for science and innovation, Fermi Lab 2012.
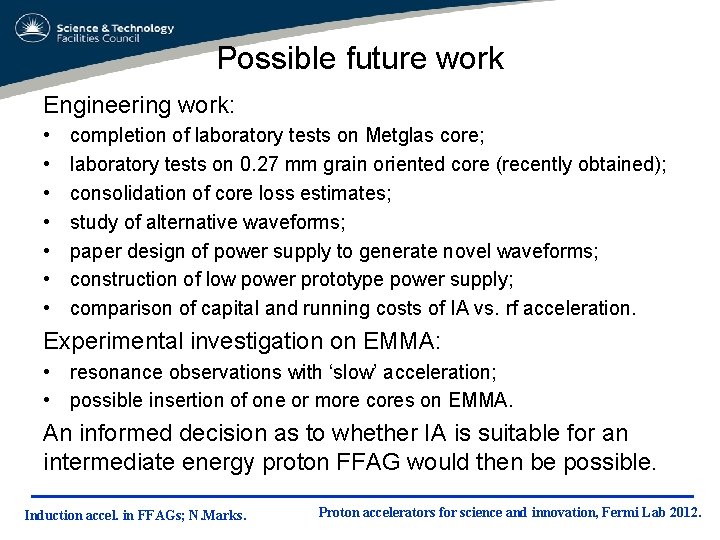
Possible future work Engineering work: • • completion of laboratory tests on Metglas core; laboratory tests on 0. 27 mm grain oriented core (recently obtained); consolidation of core loss estimates; study of alternative waveforms; paper design of power supply to generate novel waveforms; construction of low power prototype power supply; comparison of capital and running costs of IA vs. rf acceleration. Experimental investigation on EMMA: • resonance observations with ‘slow’ acceleration; • possible insertion of one or more cores on EMMA. An informed decision as to whether IA is suitable for an intermediate energy proton FFAG would then be possible. Induction accel. in FFAGs; N. Marks. Proton accelerators for science and innovation, Fermi Lab 2012.
Centripetal acceleration tangential acceleration
Angular velocity to linear velocity
Radial acceleration
Kinetic angular energy
Centripital acceleration
World population prospects
Elysian prospects
Prospects preposition
Quantum computing progress and prospects
Prospects of agriculture in bangladesh
Multiplikation med uppställning
Delegerande ledarstil
Kassaregister ideell förening
Toppslätskivling dos
Redogör för vad psykologi är
Borra hål för knoppar
Bris för vuxna
Bra mat för unga idrottare
Offentlig förvaltning
Teckenspråk minoritetsspråk argument
Ledarskapsteorier
Datorkunskap för nybörjare
Plagg i gamla rom
Humanitr
Rita perspektiv
Ministerstyre för och nackdelar
Nationell inriktning för artificiell intelligens
Claes martinsson
Sju principer för tillitsbaserad styrning
Gumman cirkel sång
Klassen rim
Nyckelkompetenser för livslångt lärande
Modell för handledningsprocess
Personlig tidbok fylla i
Orubbliga rättigheter