Preliminary Planning for an International Mars Sample Return
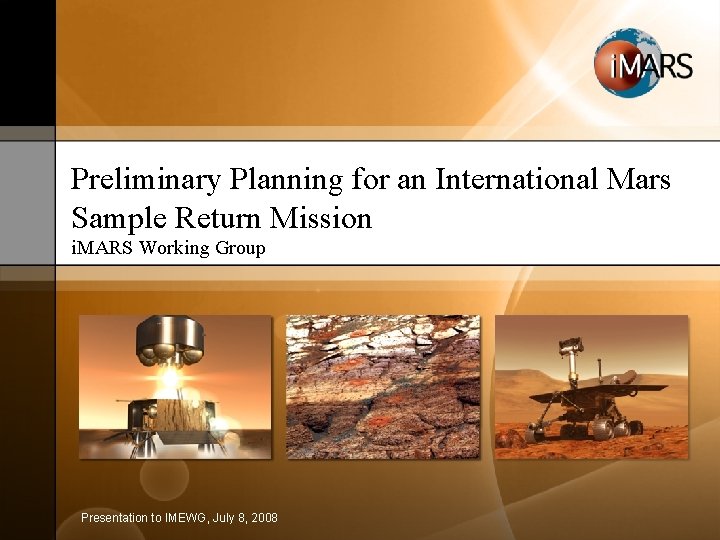
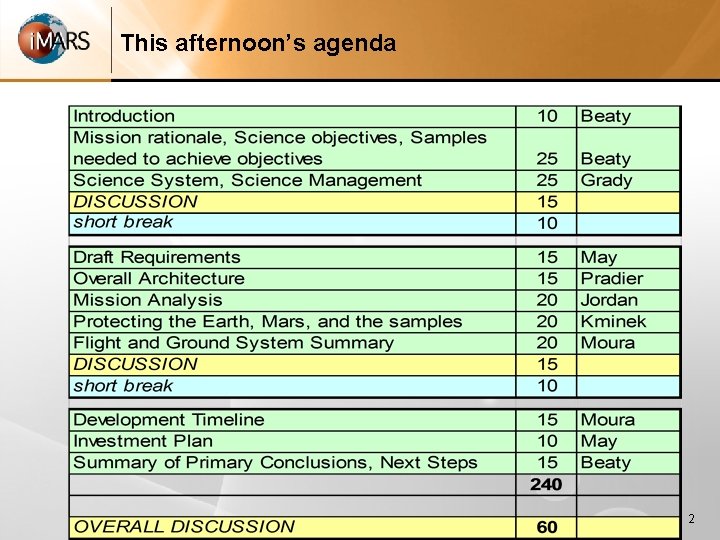
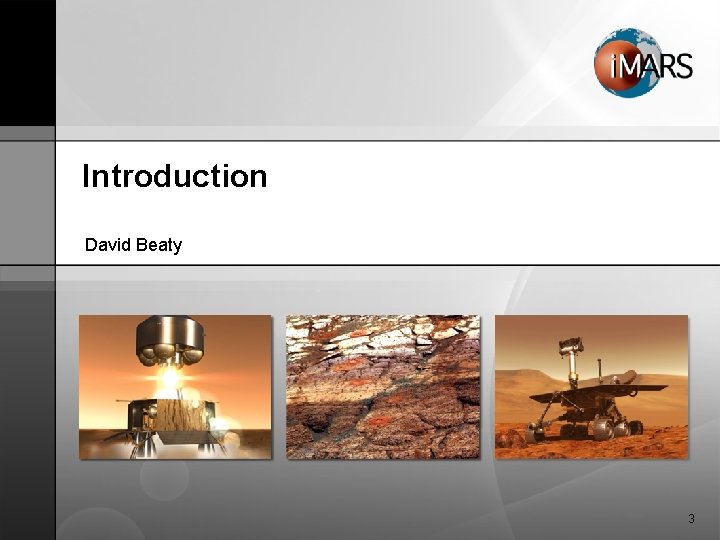
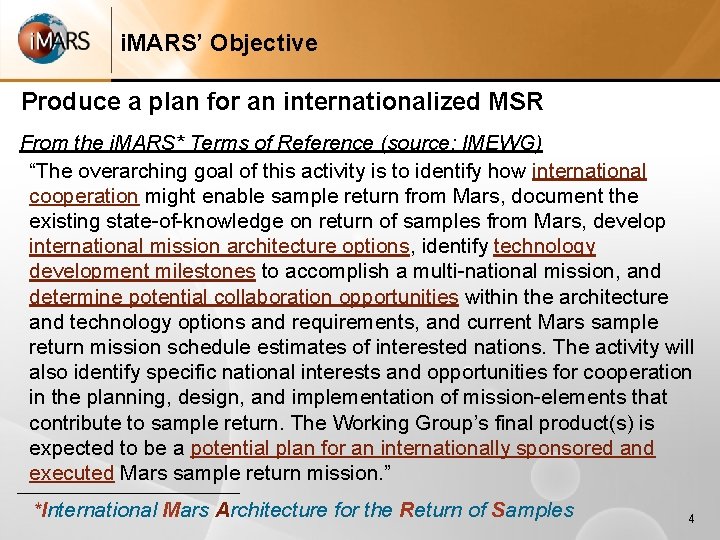
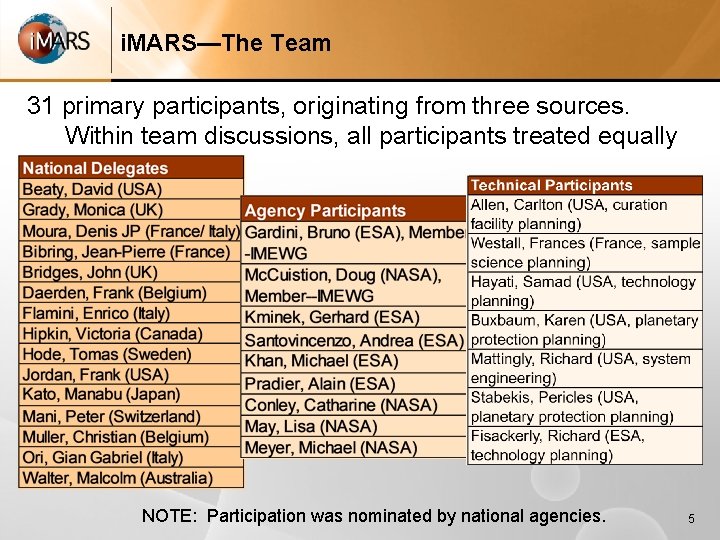
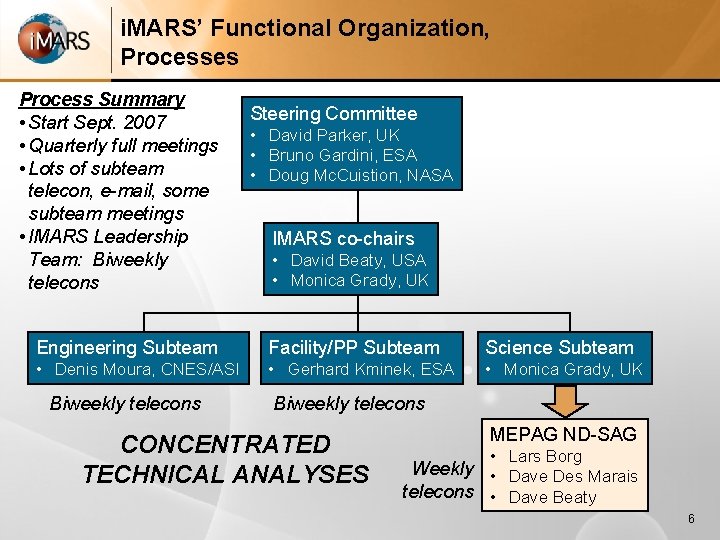
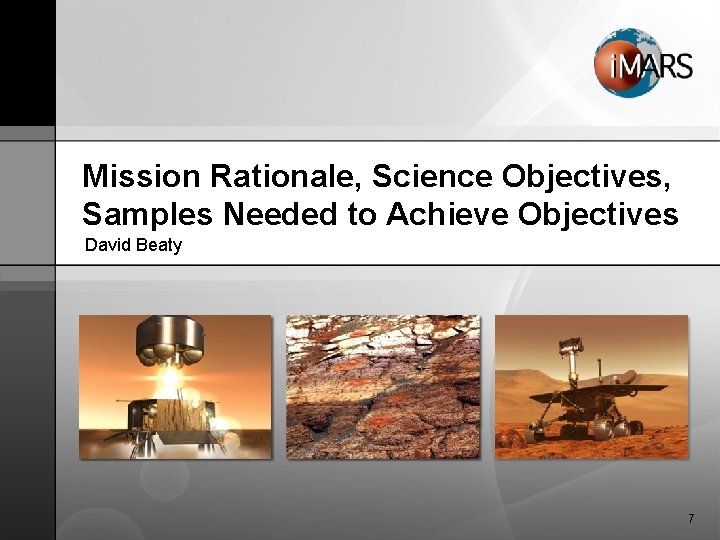
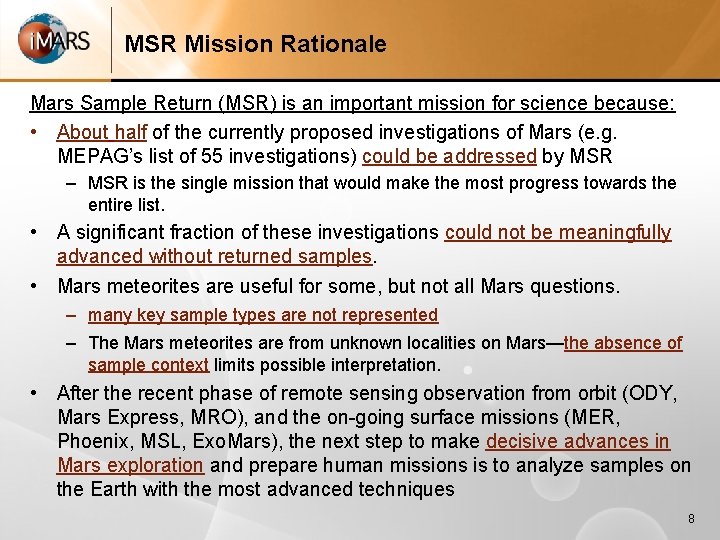
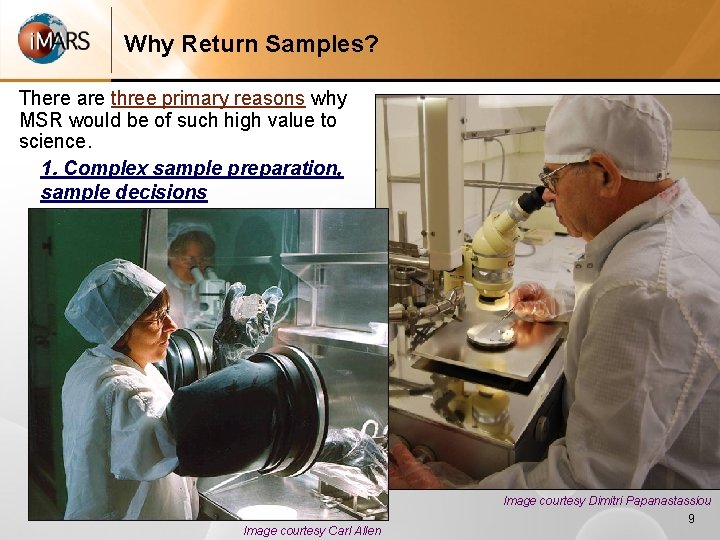
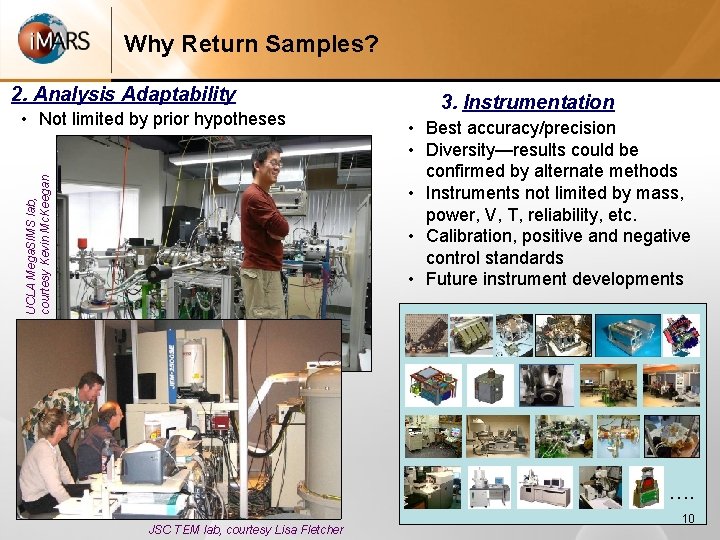
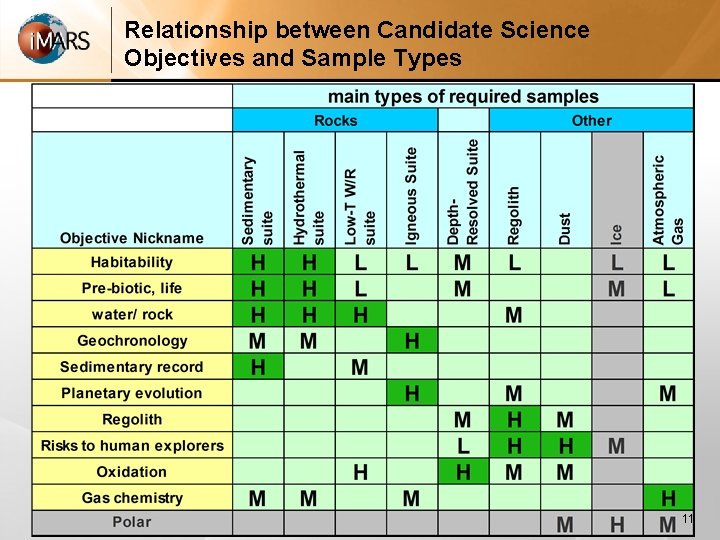
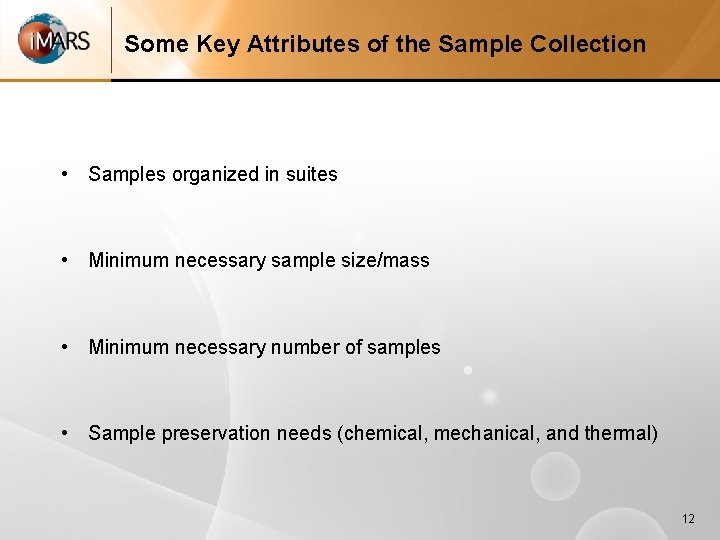
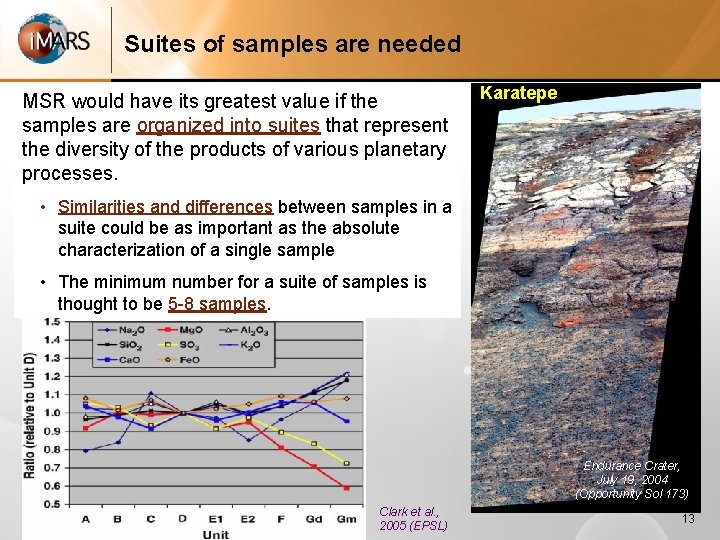
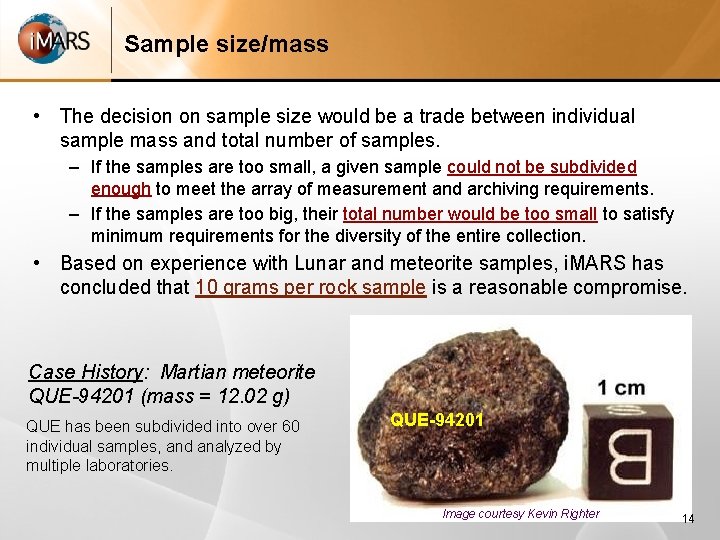
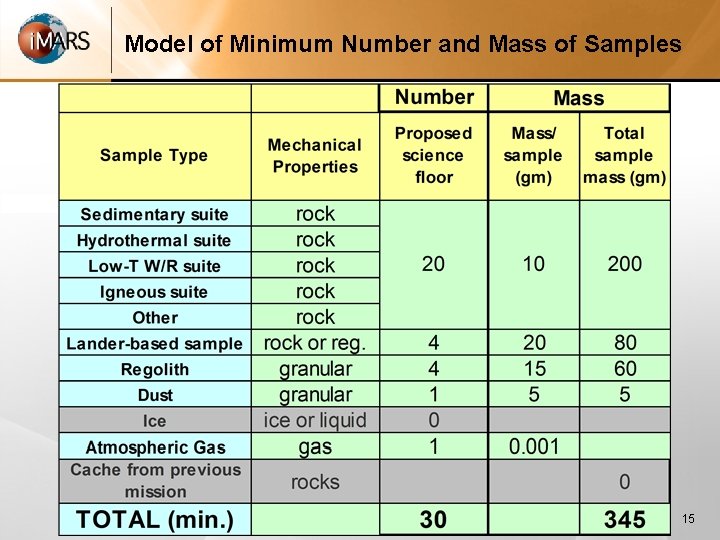
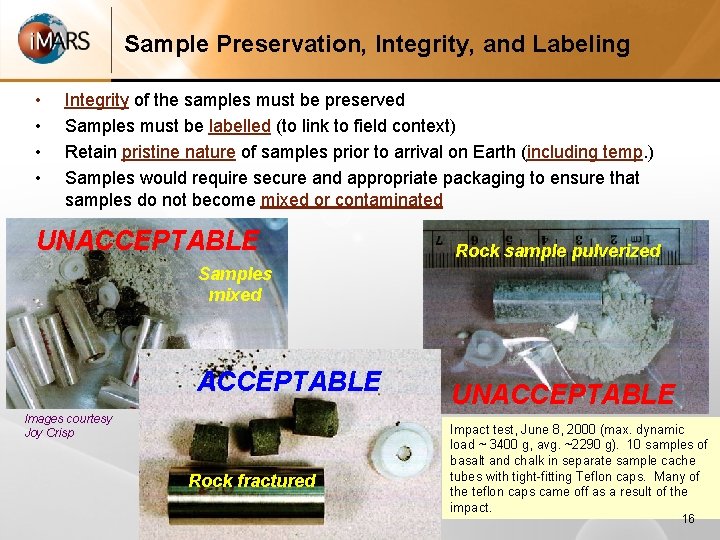
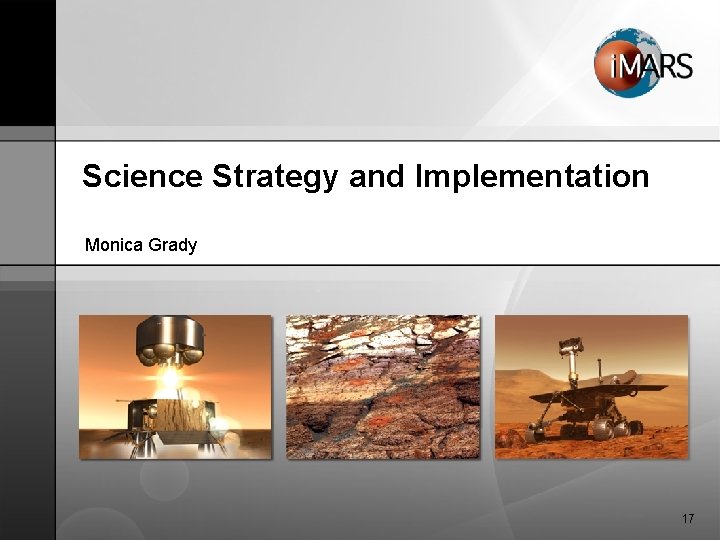
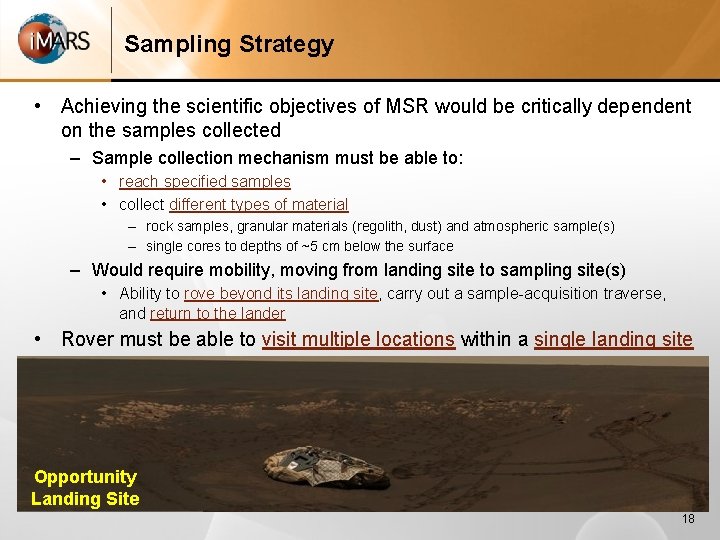
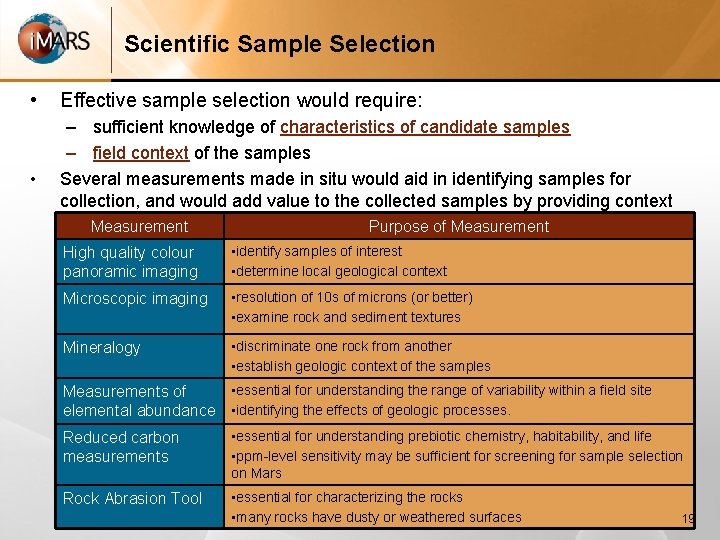
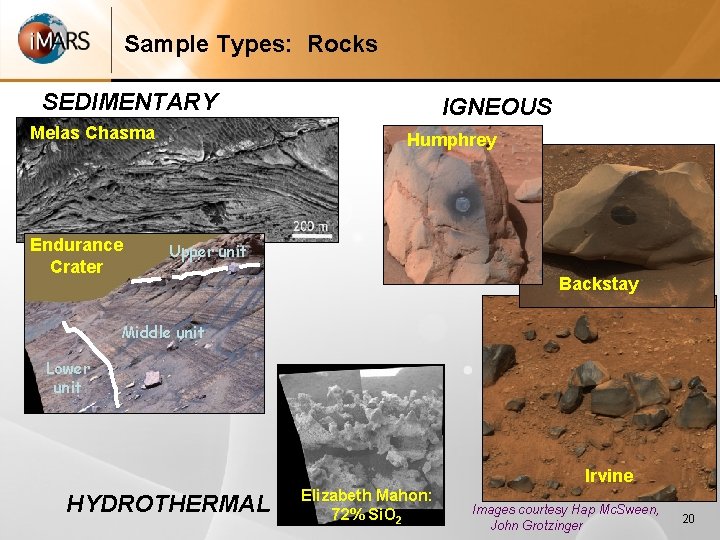
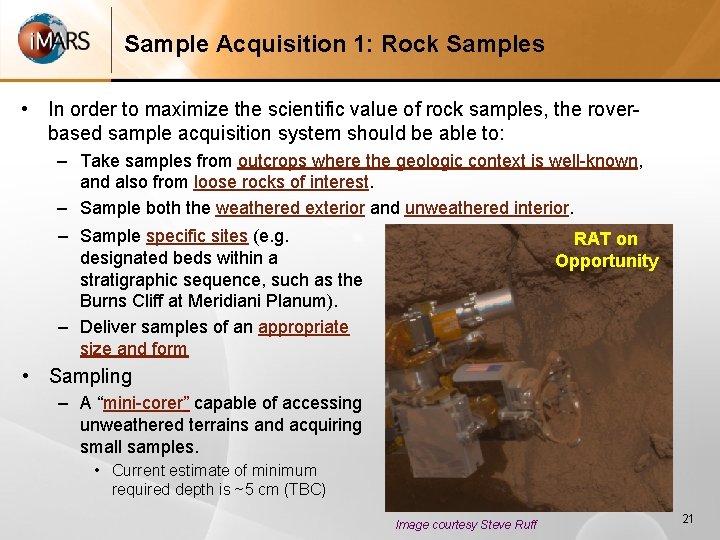
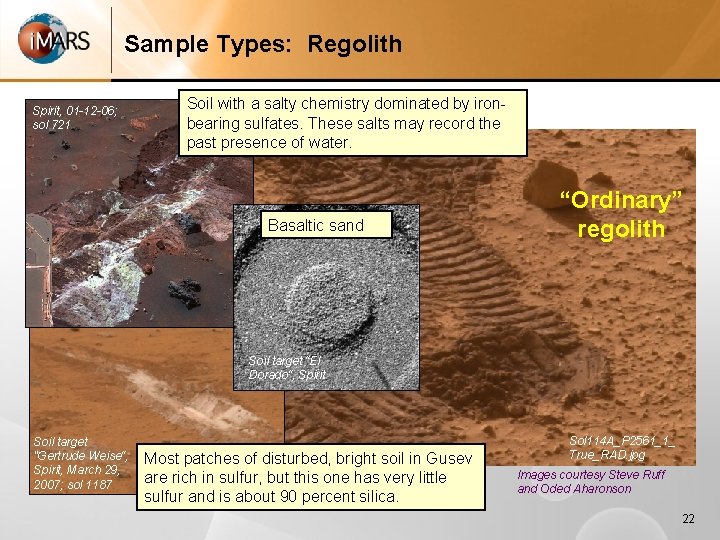
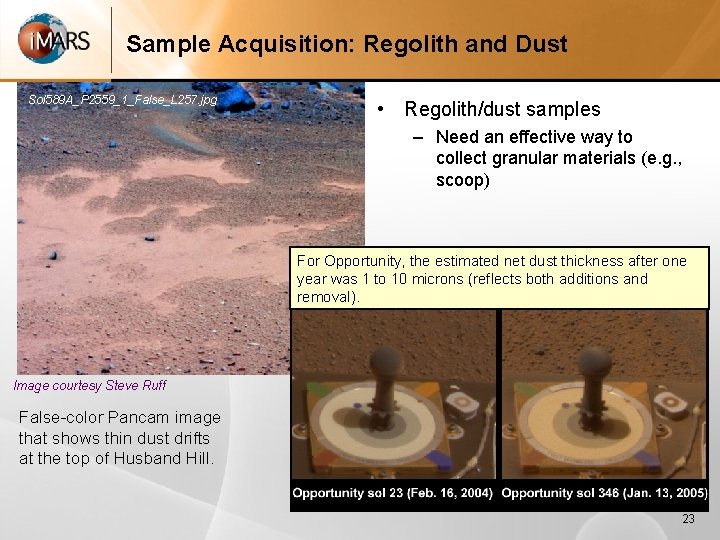
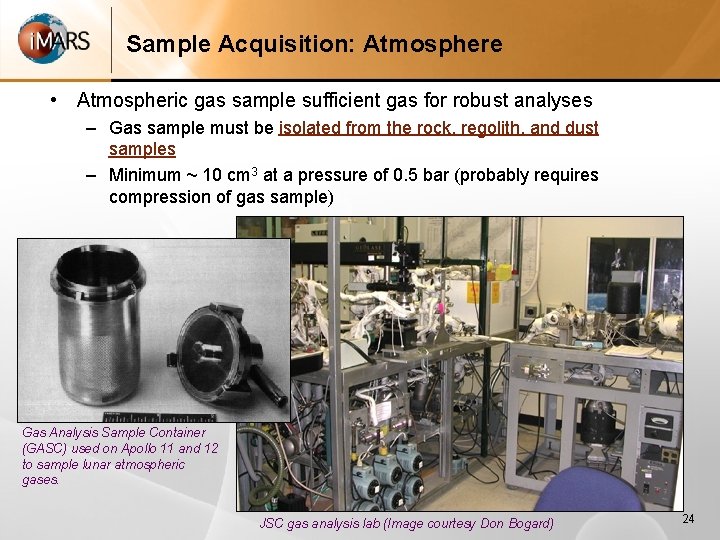
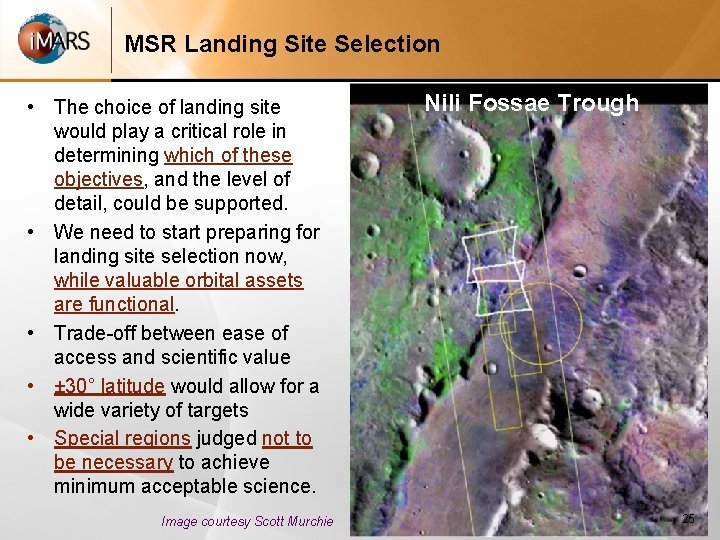
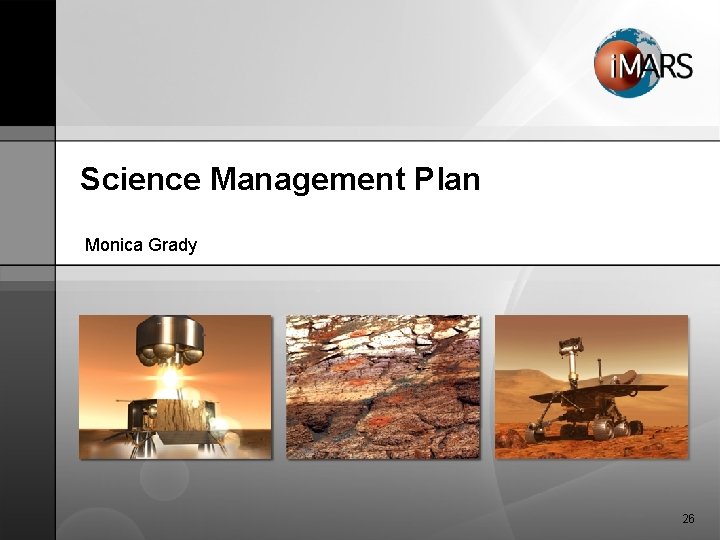
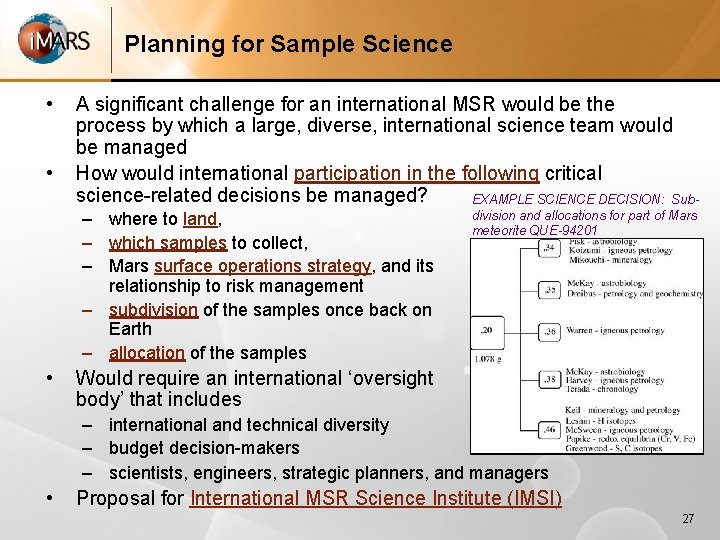
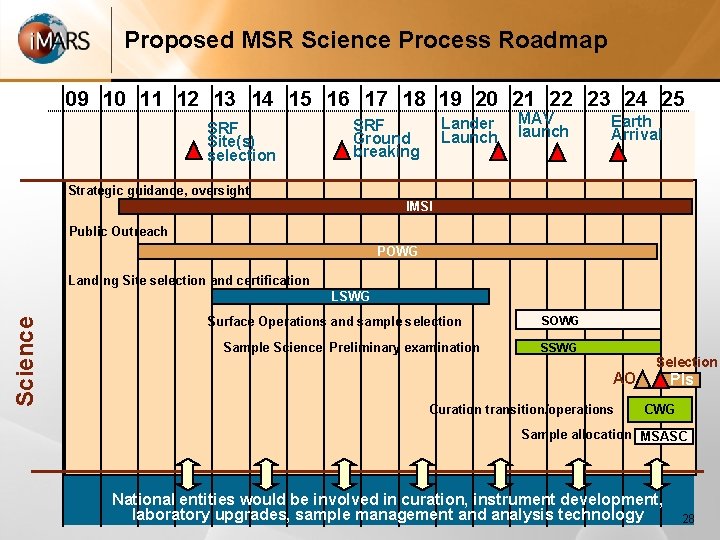
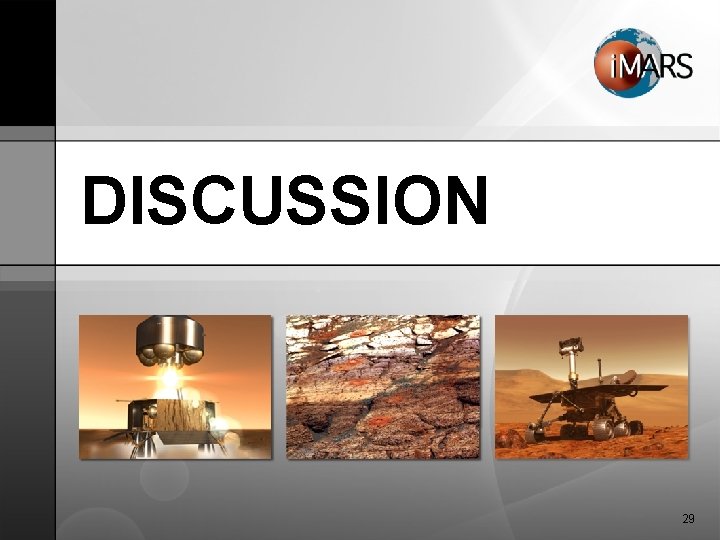
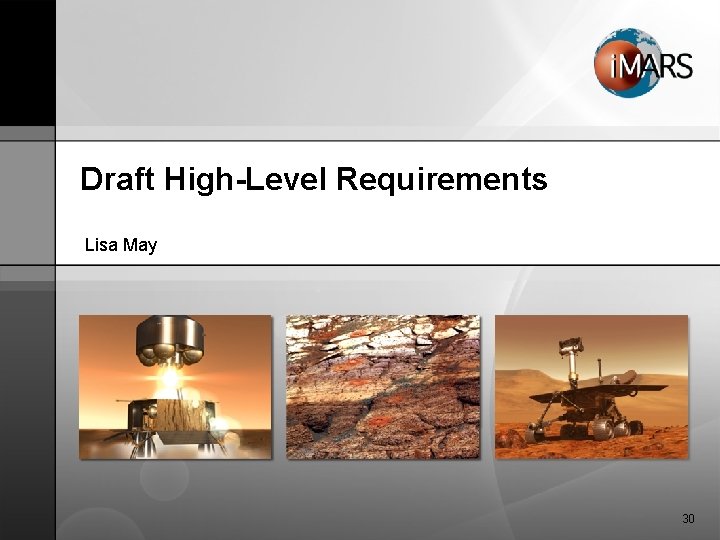
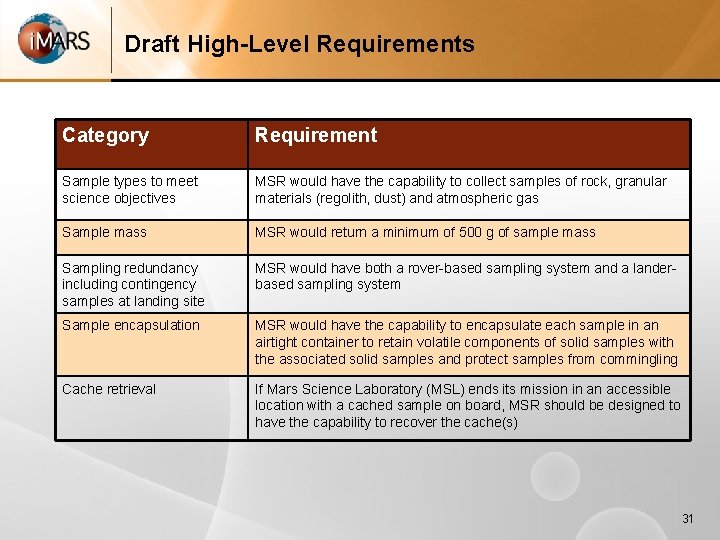
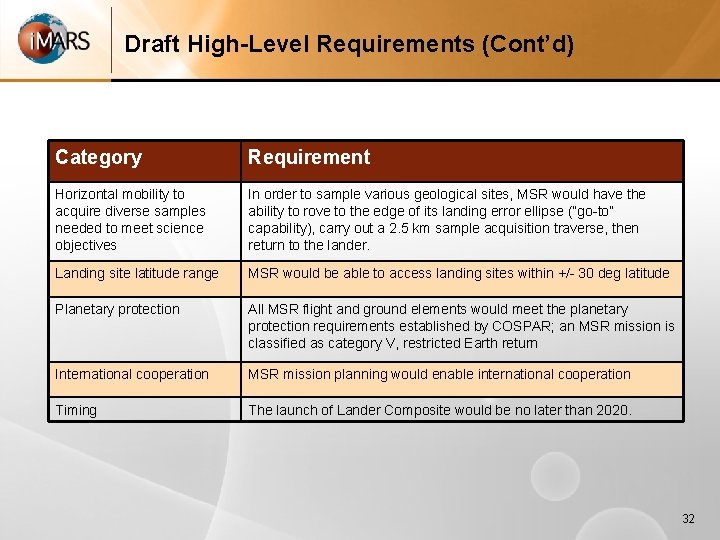
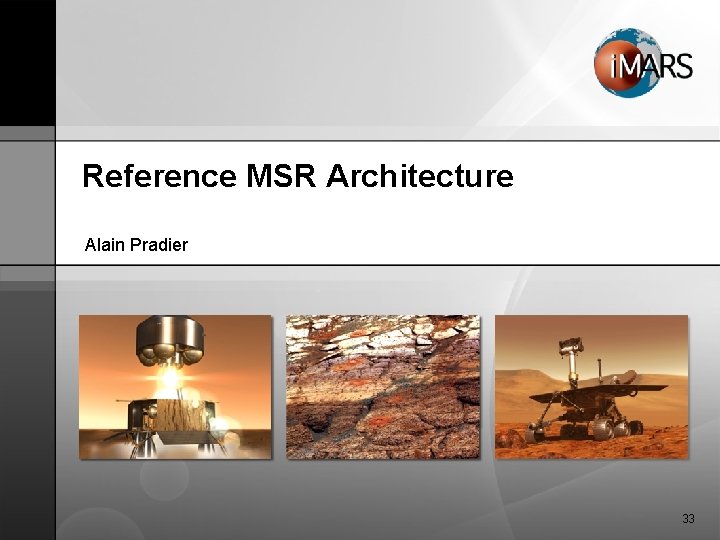
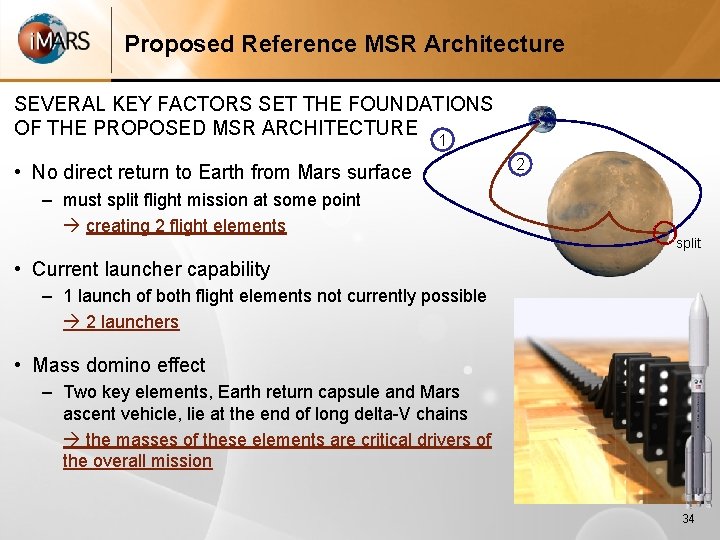
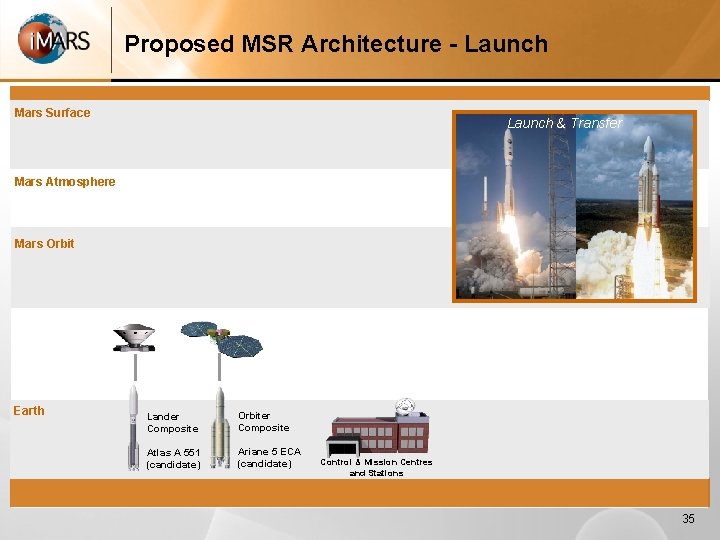
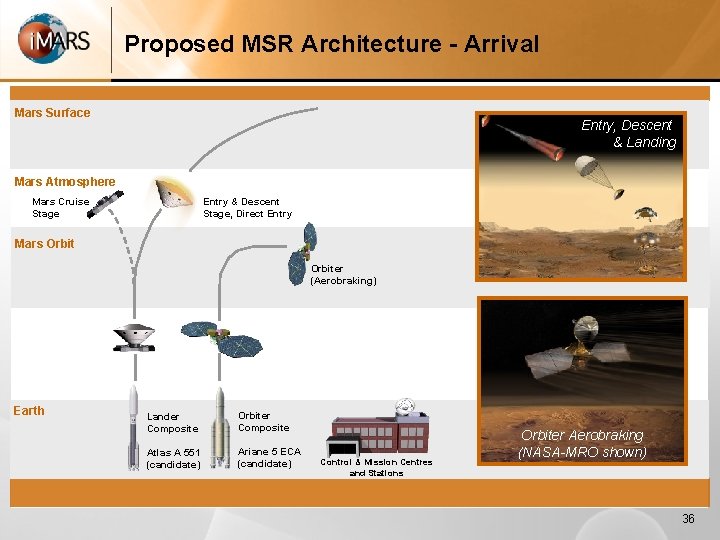
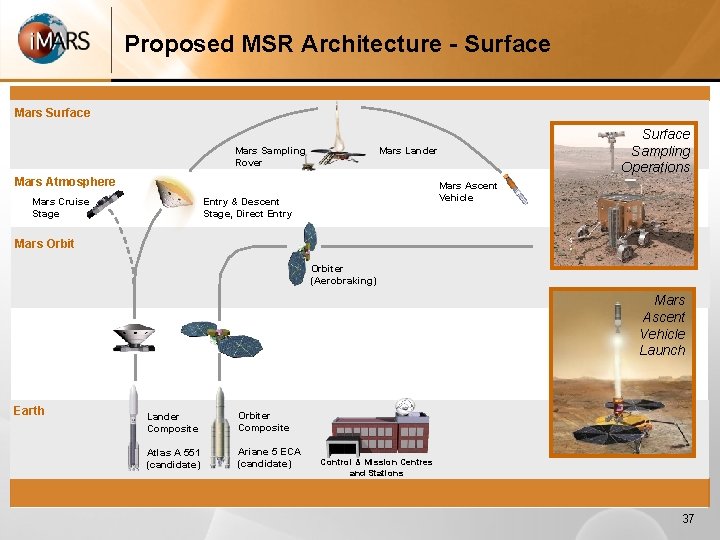
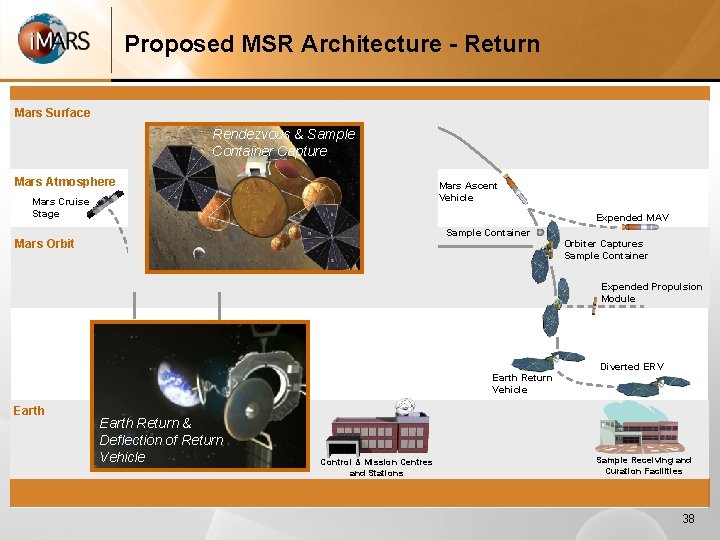
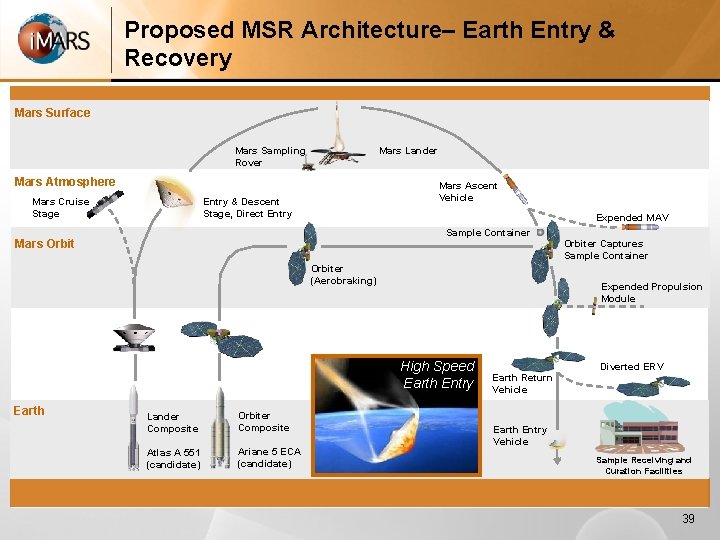
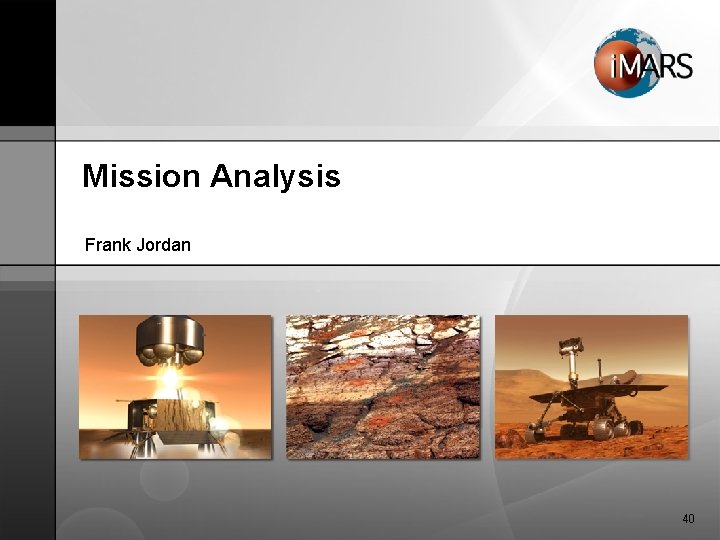
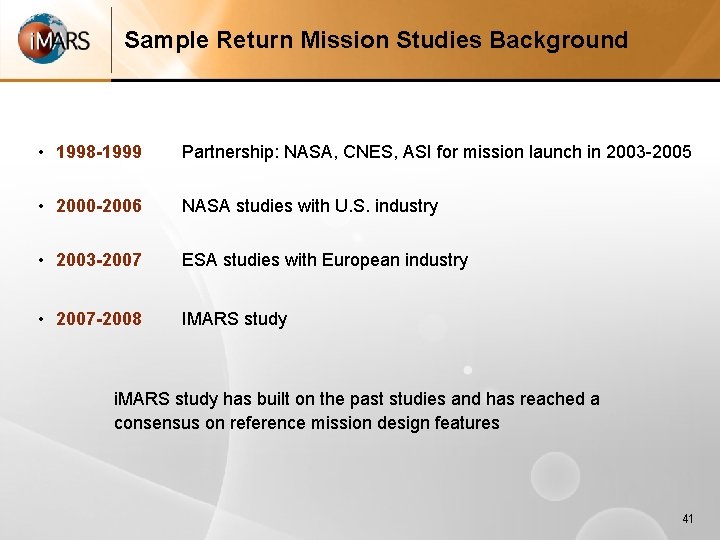
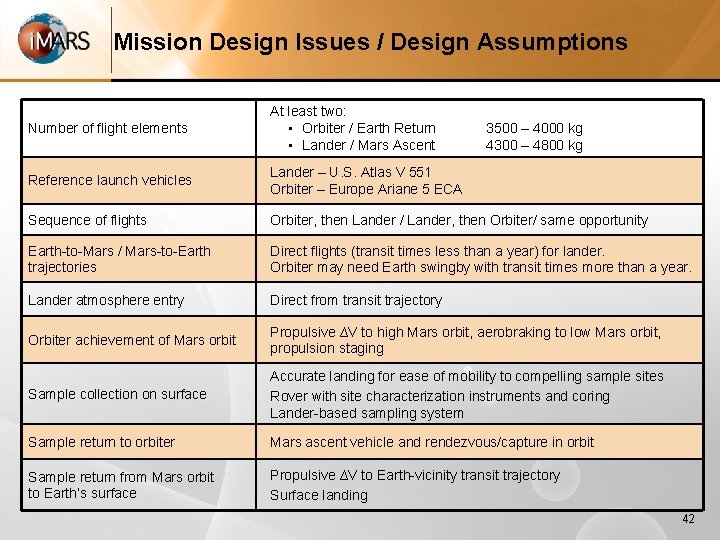
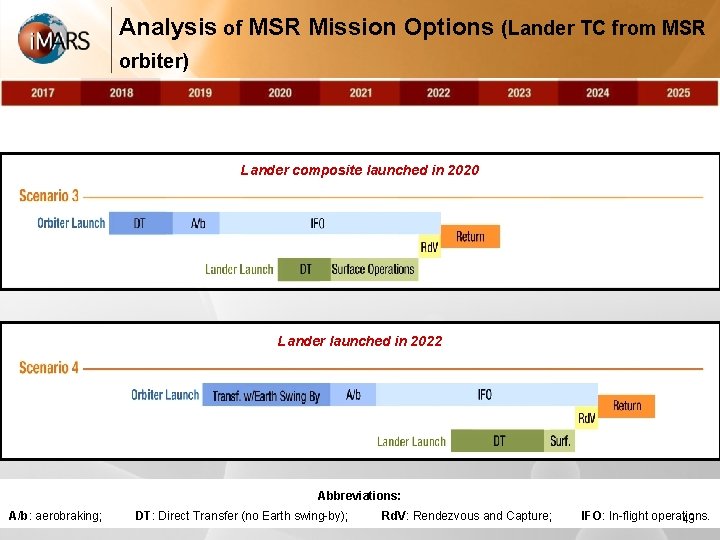
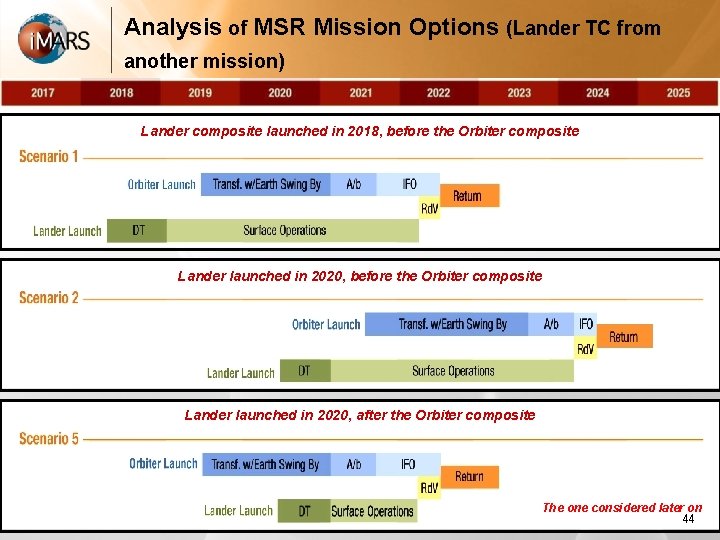
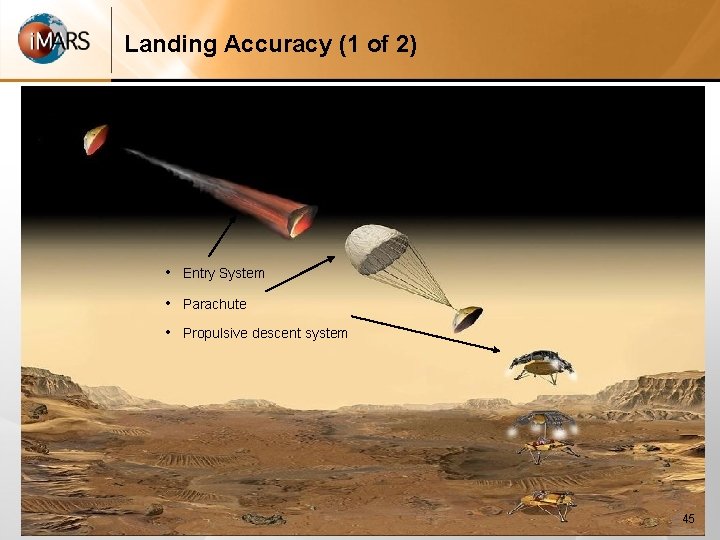
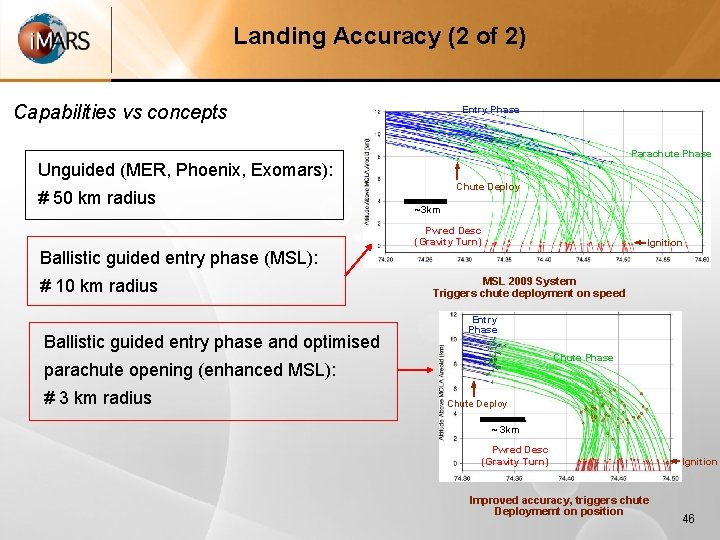
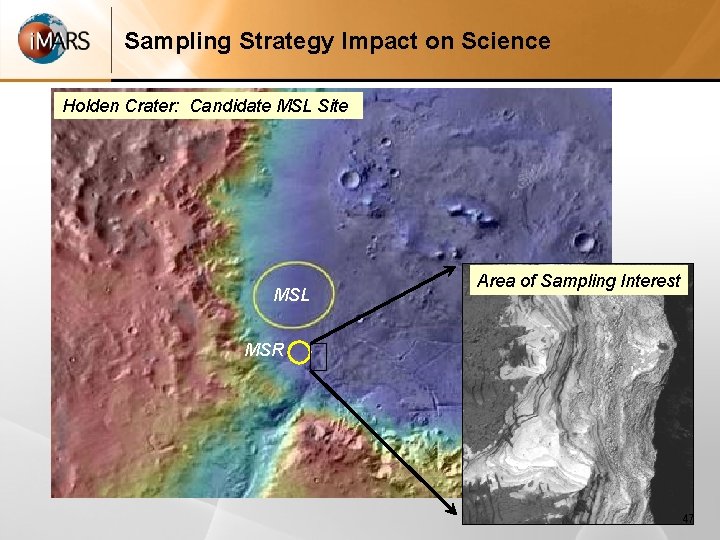
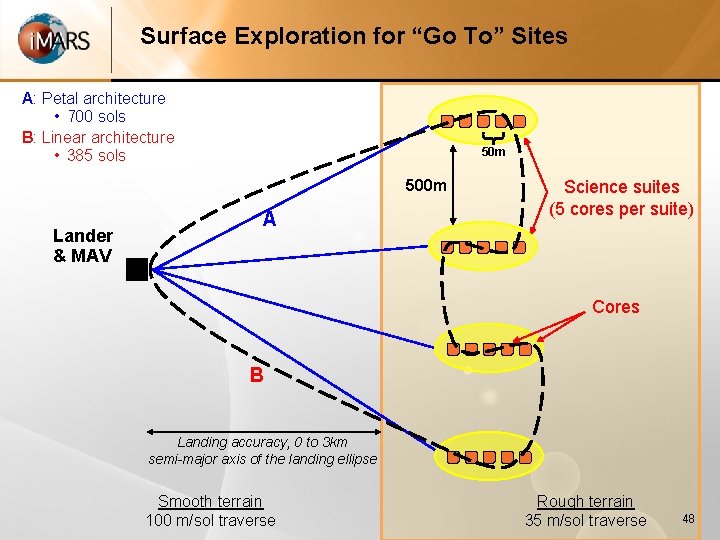
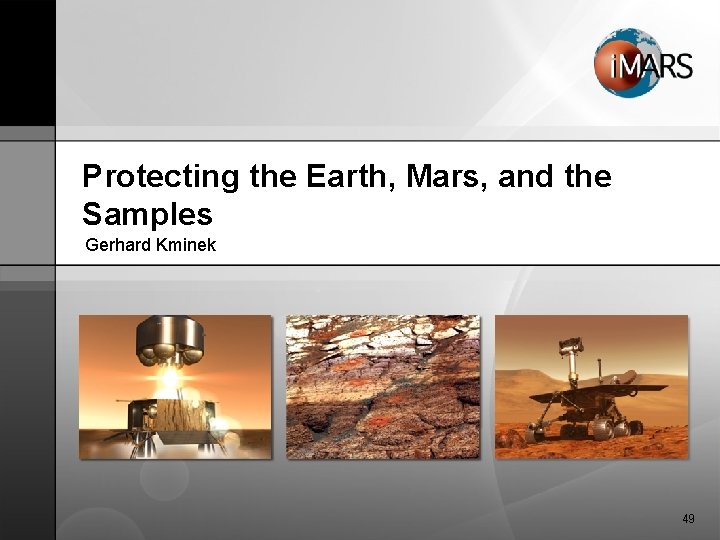
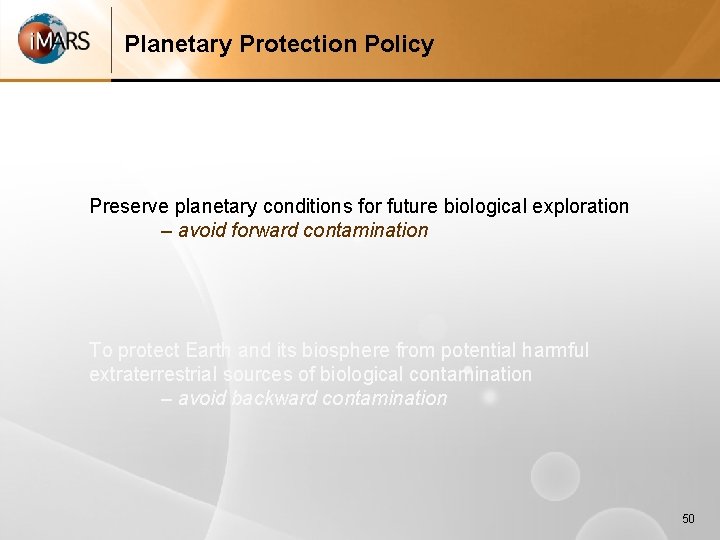
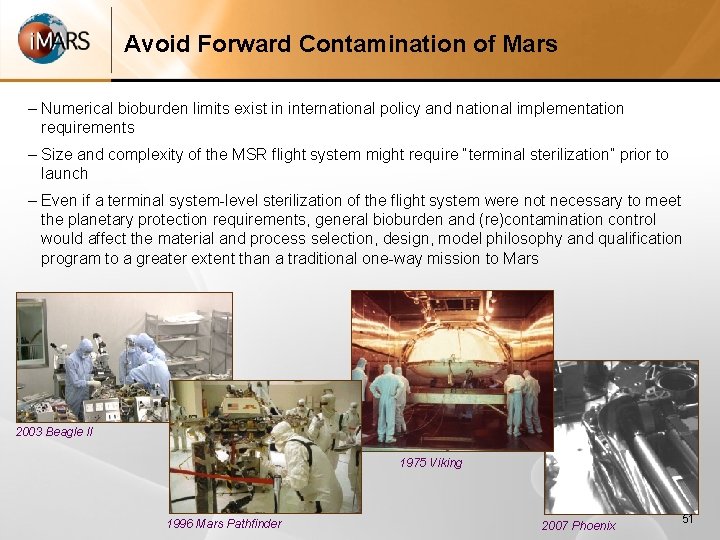
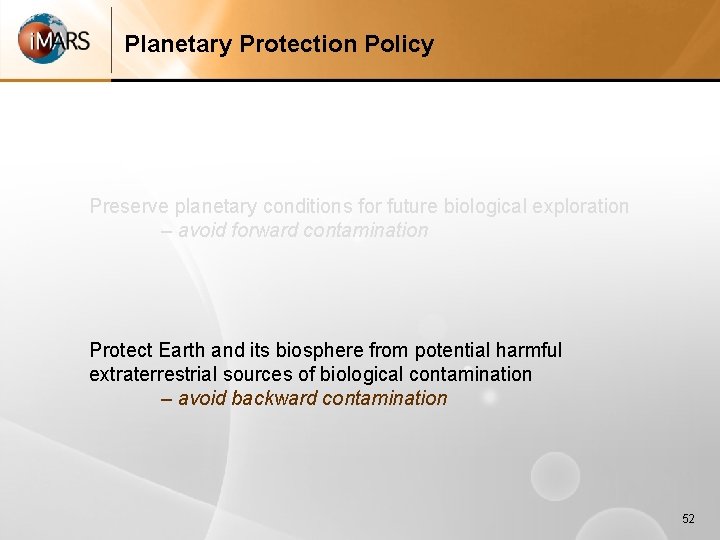
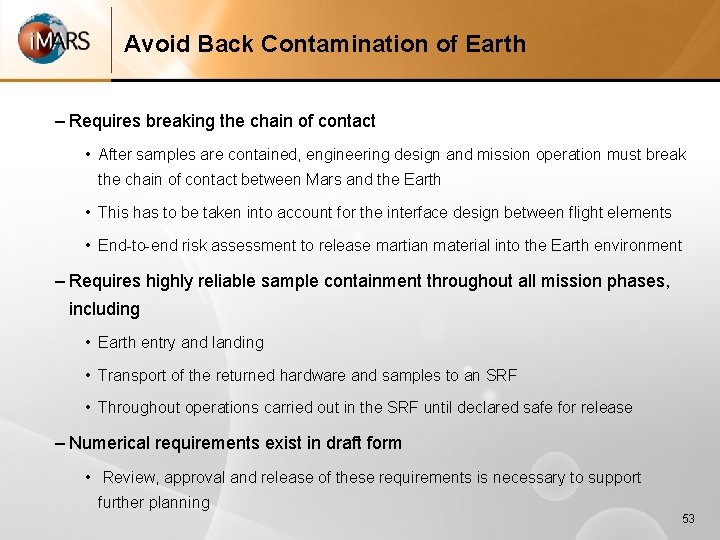
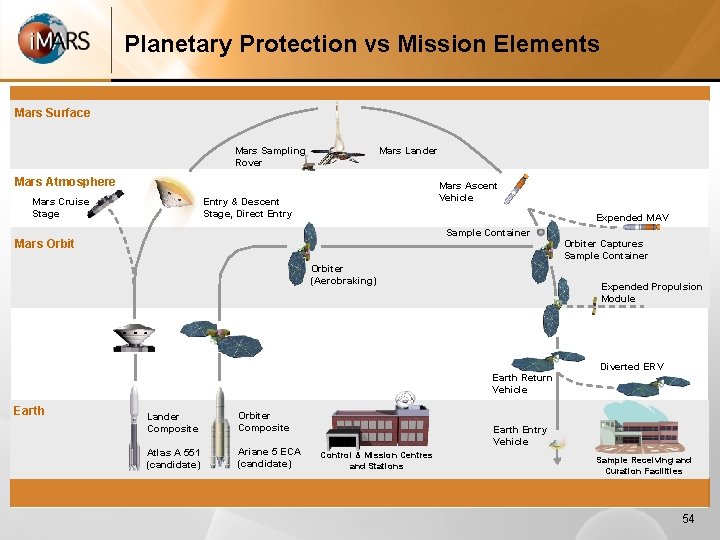
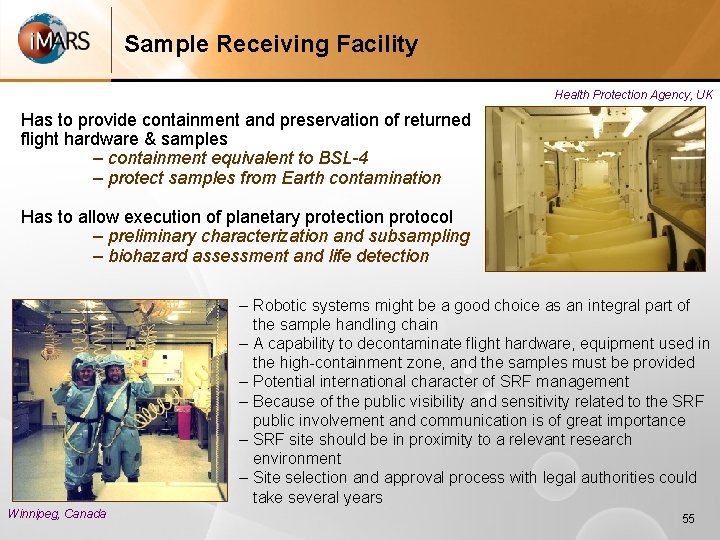
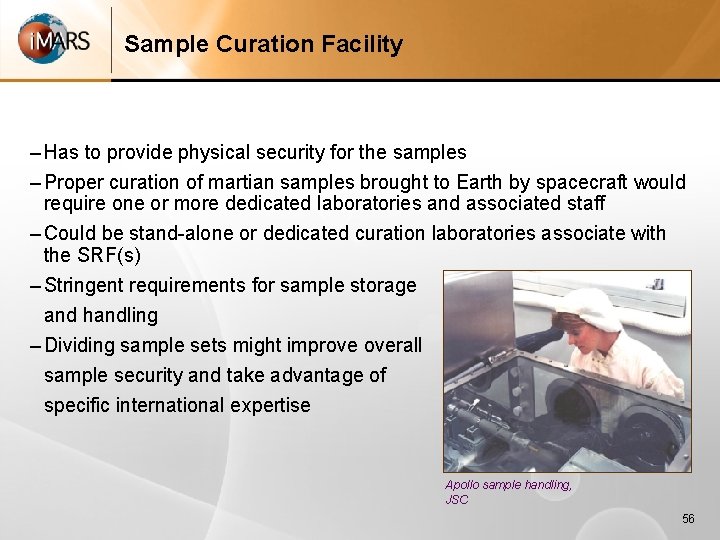
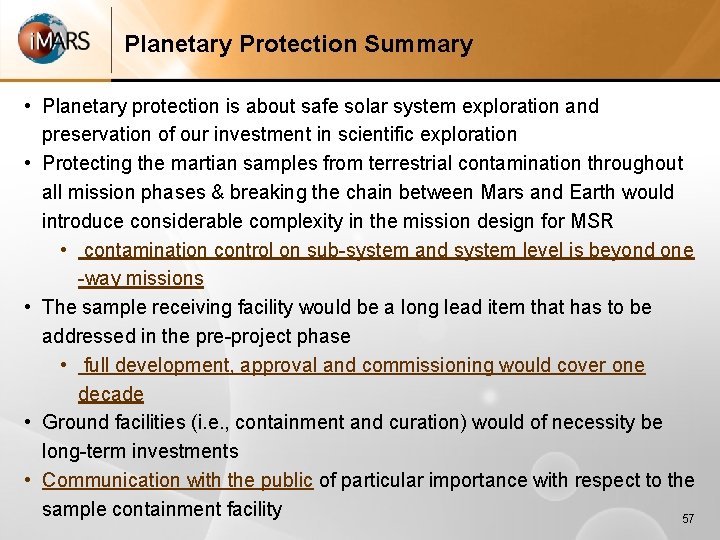
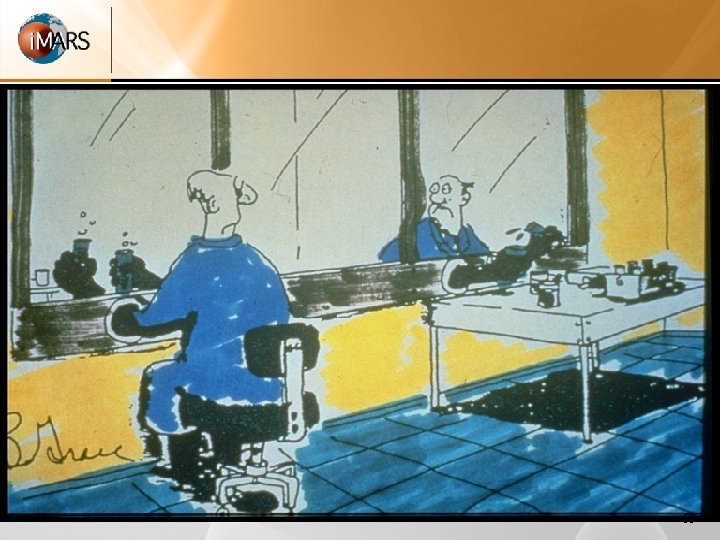
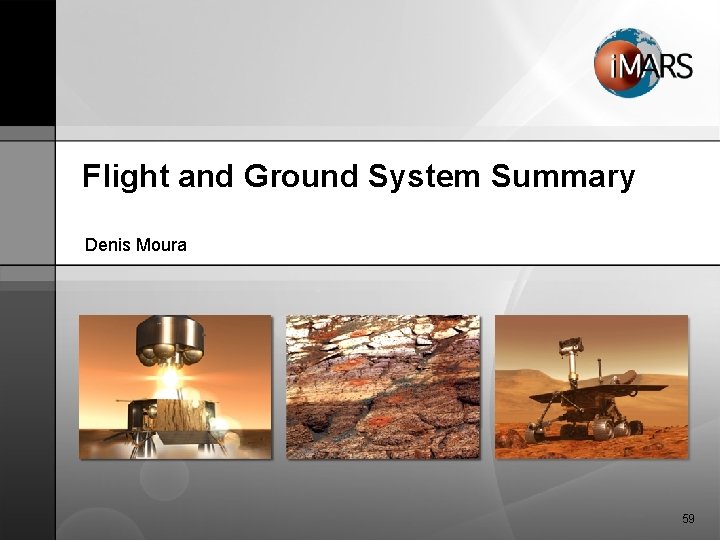
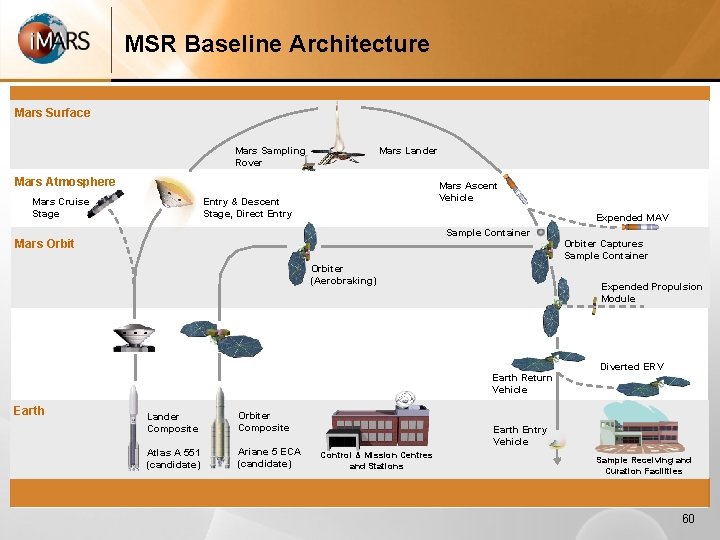
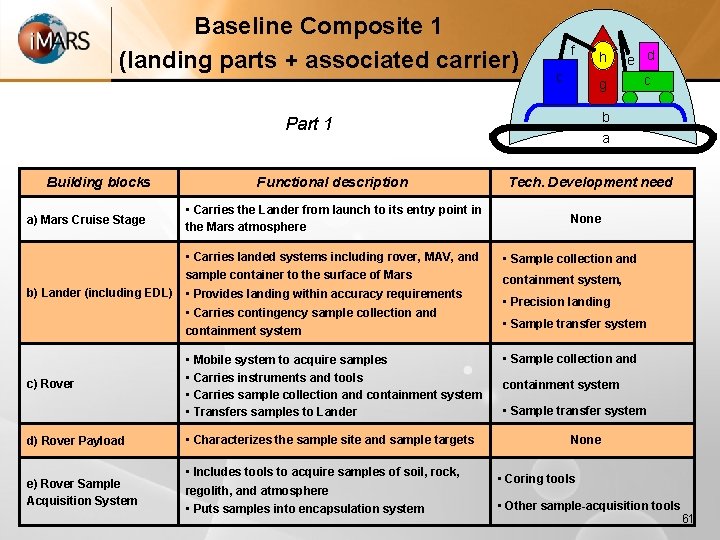
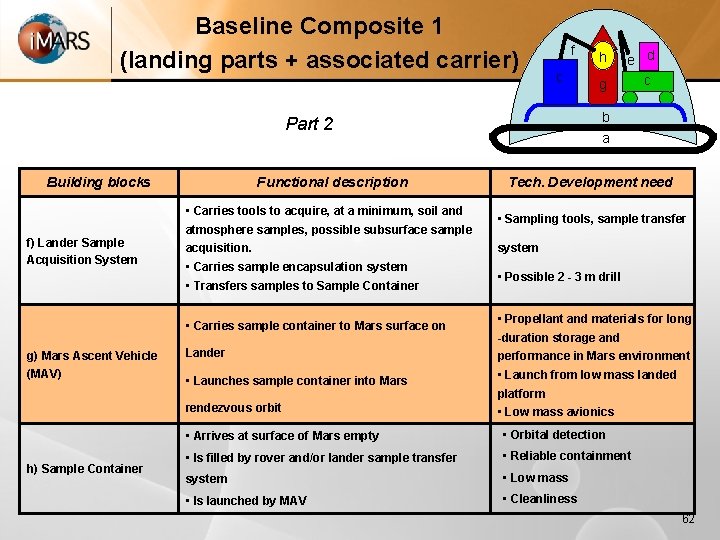
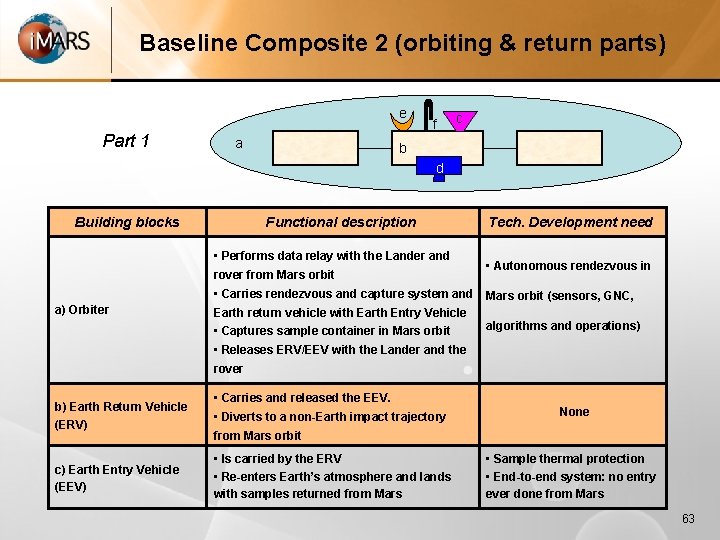
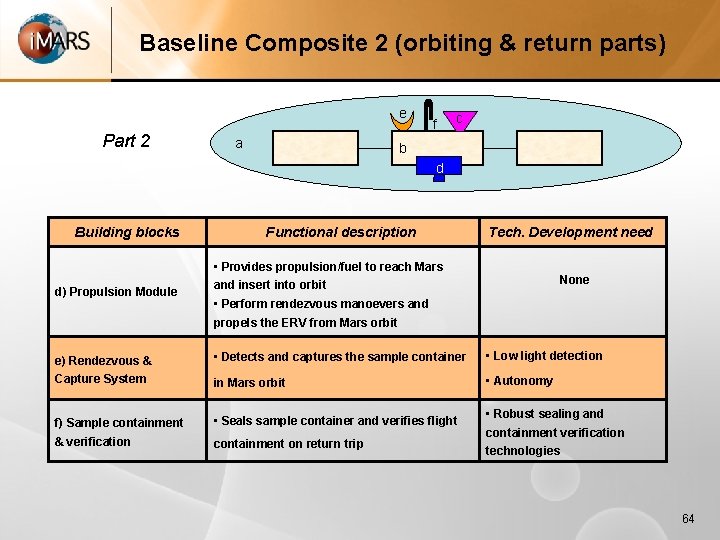
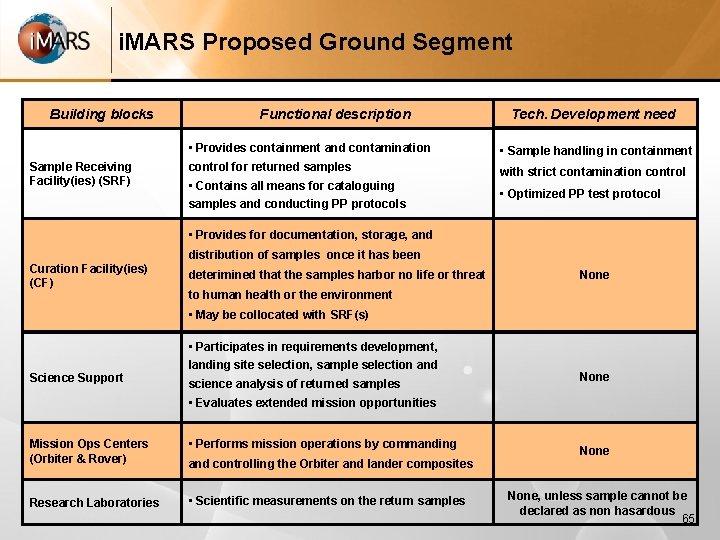
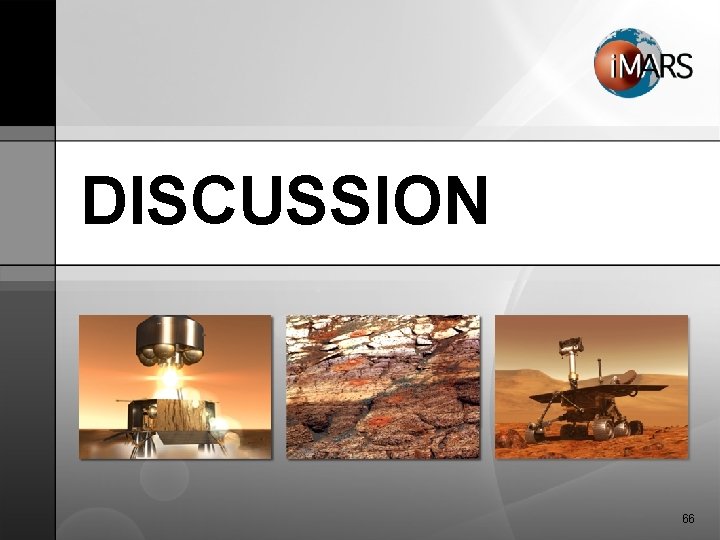
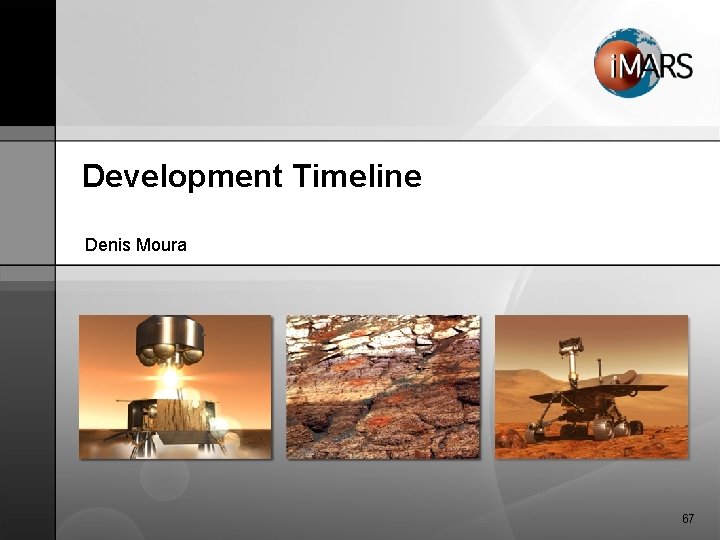
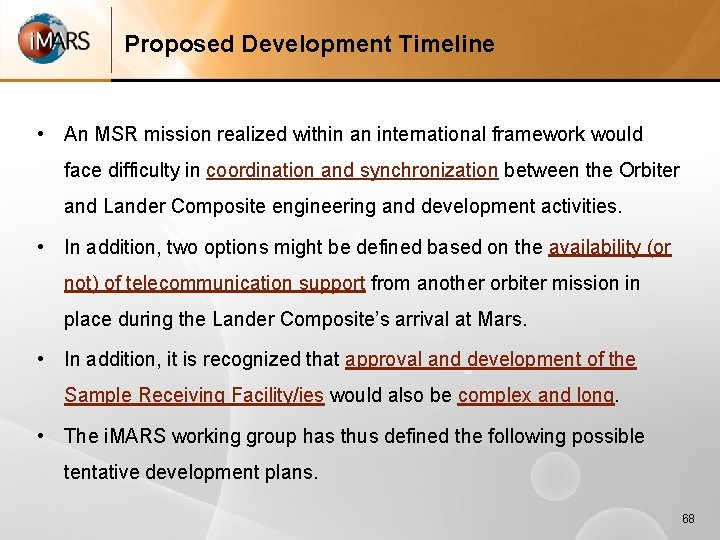
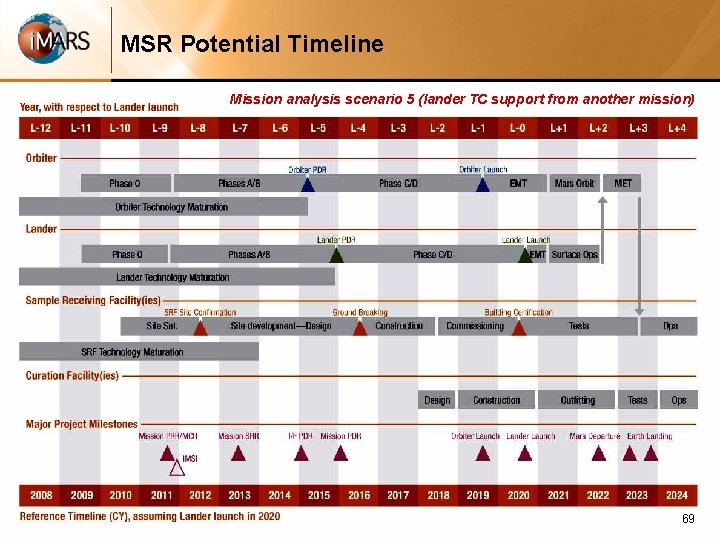
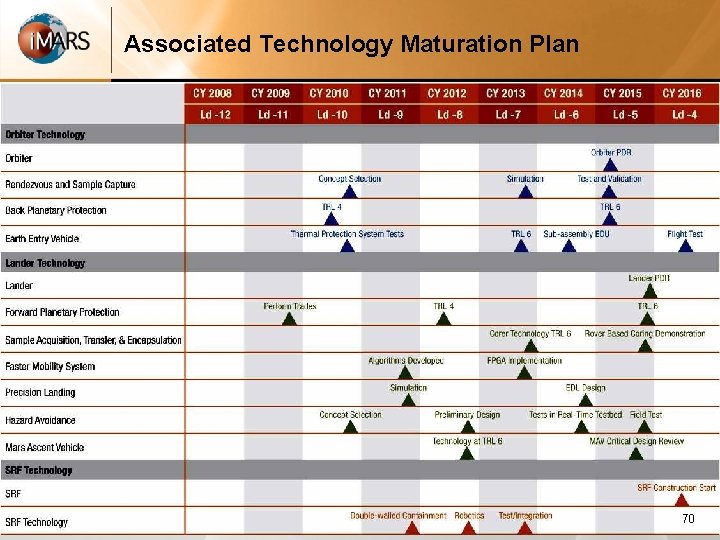
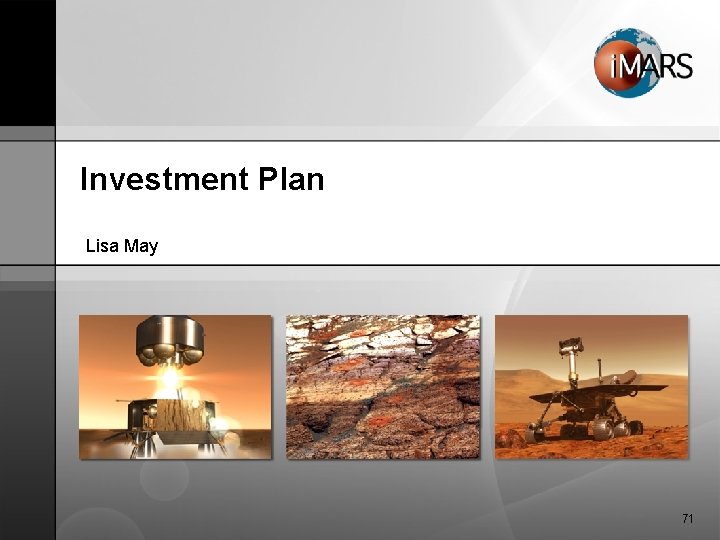
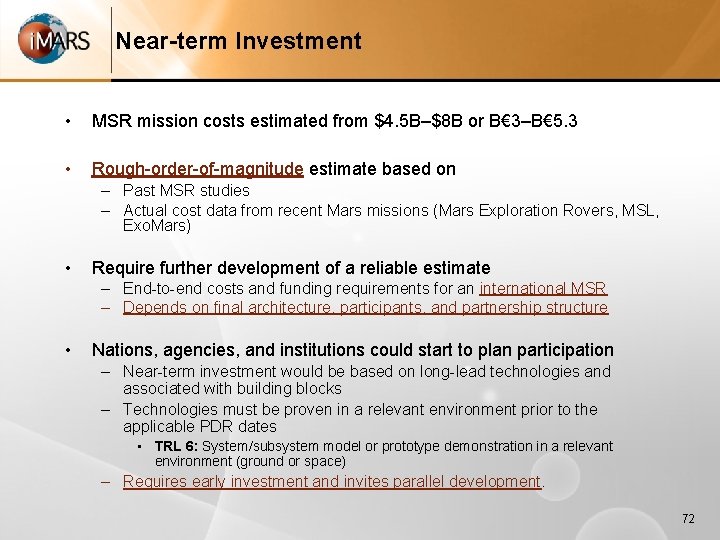
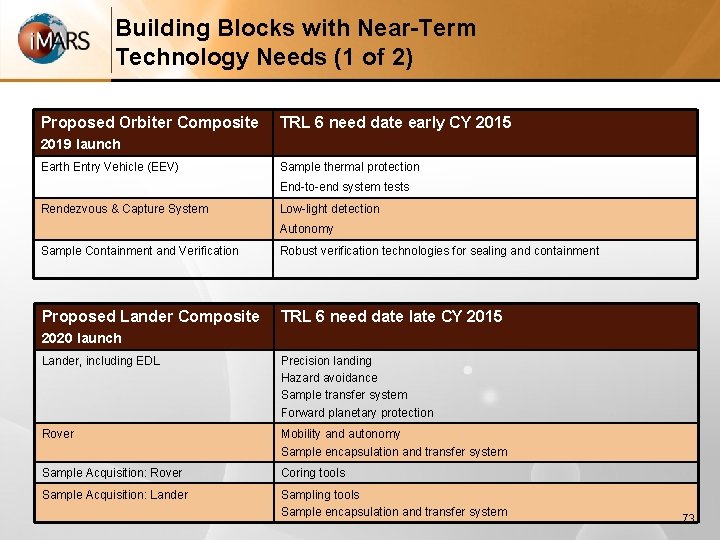
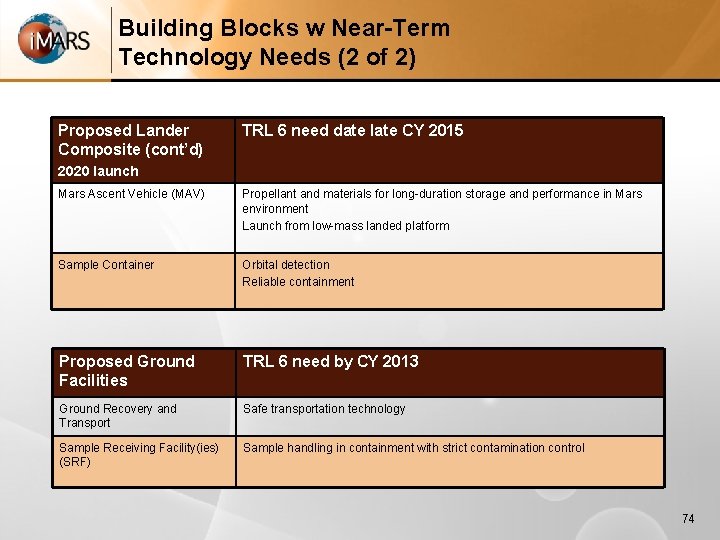
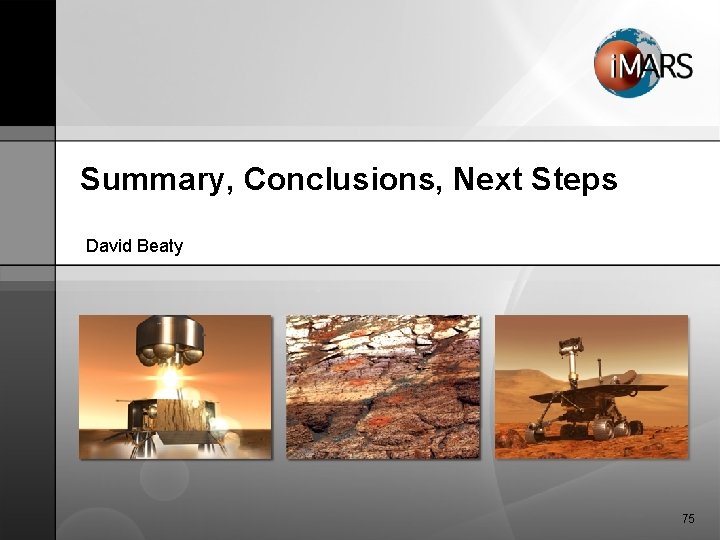
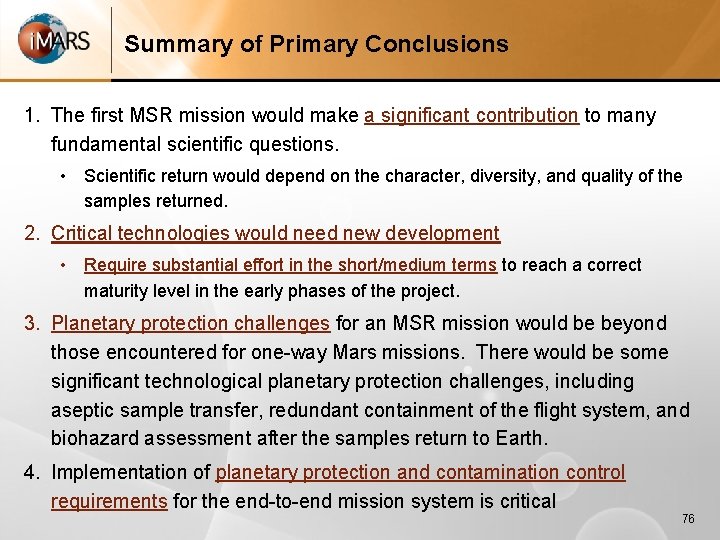
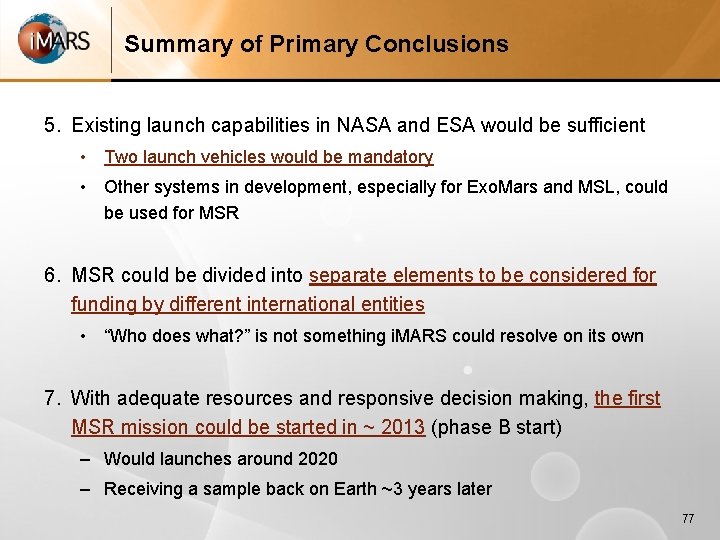
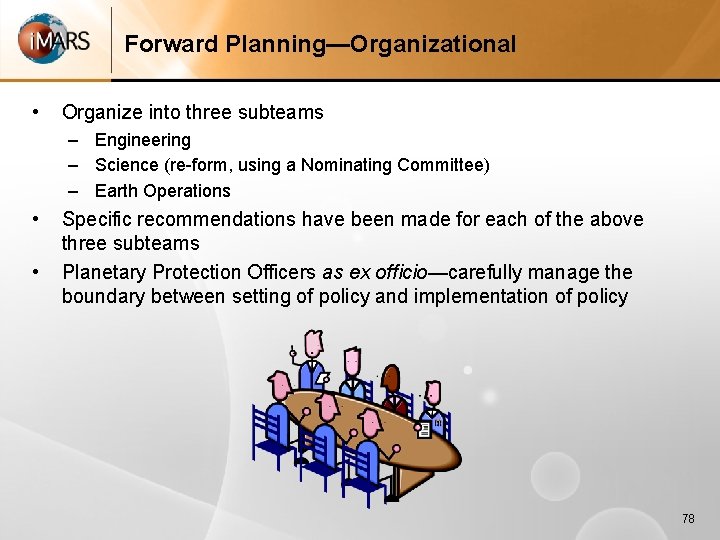
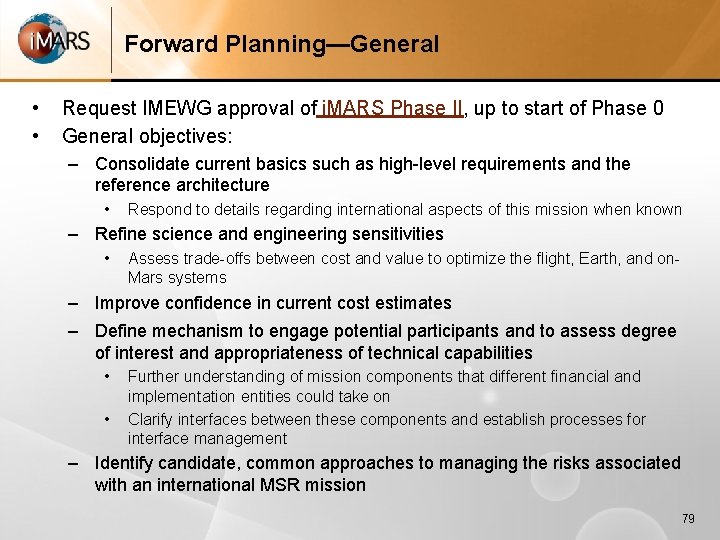
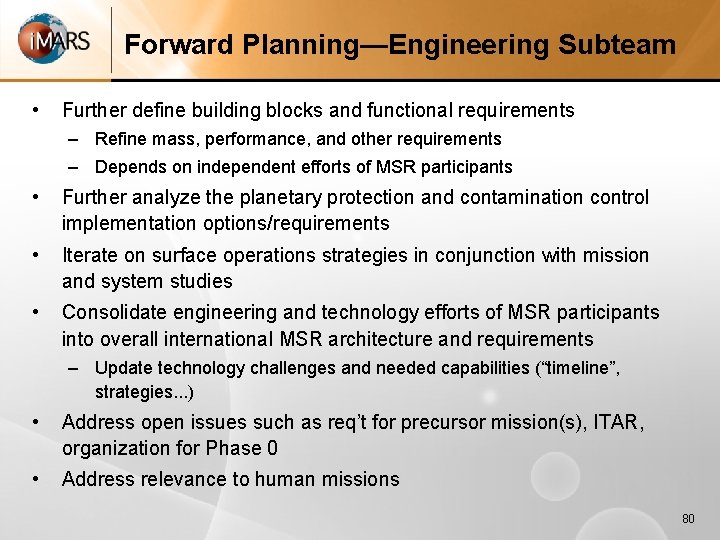
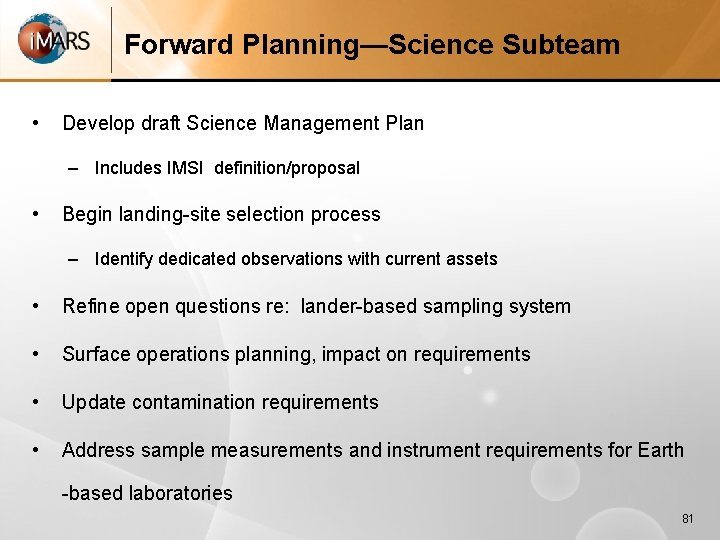
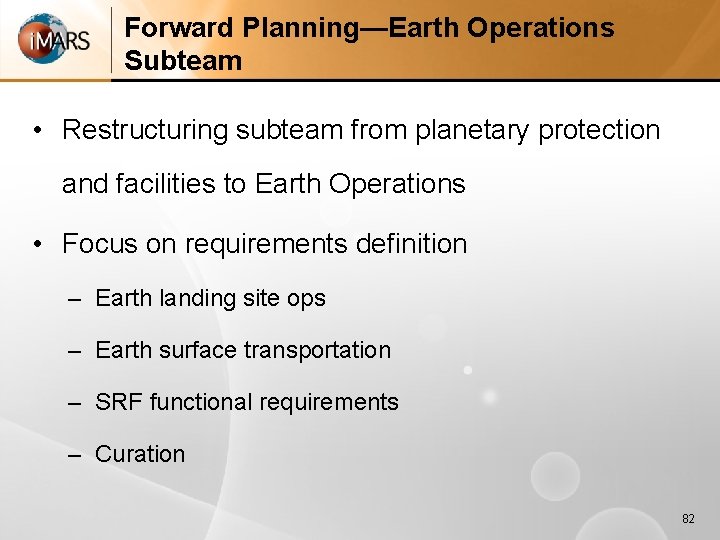
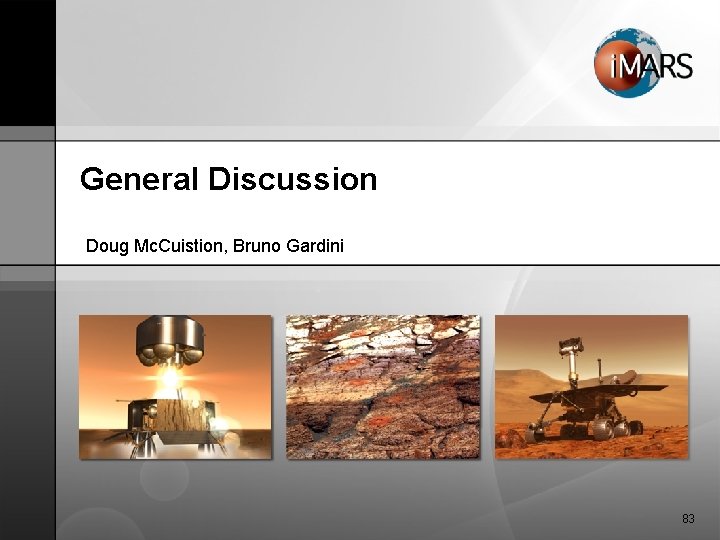
- Slides: 83
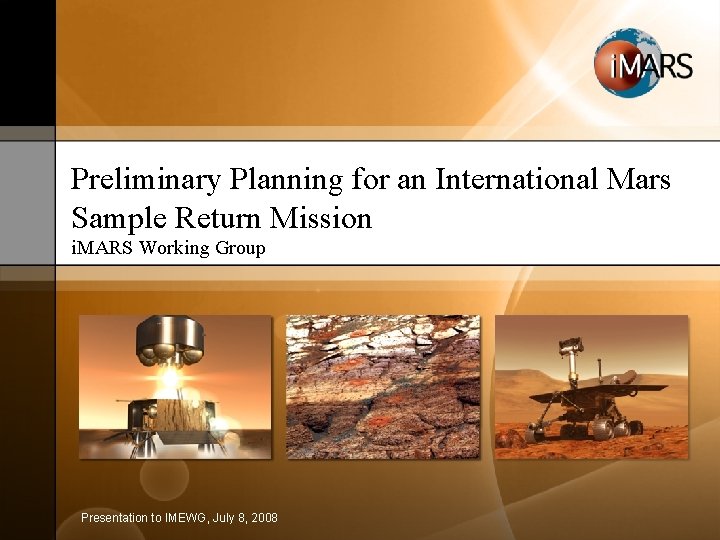
Preliminary Planning for an International Mars Sample Return Mission i. MARS Working Group Presentation to IMEWG, July 8, 2008
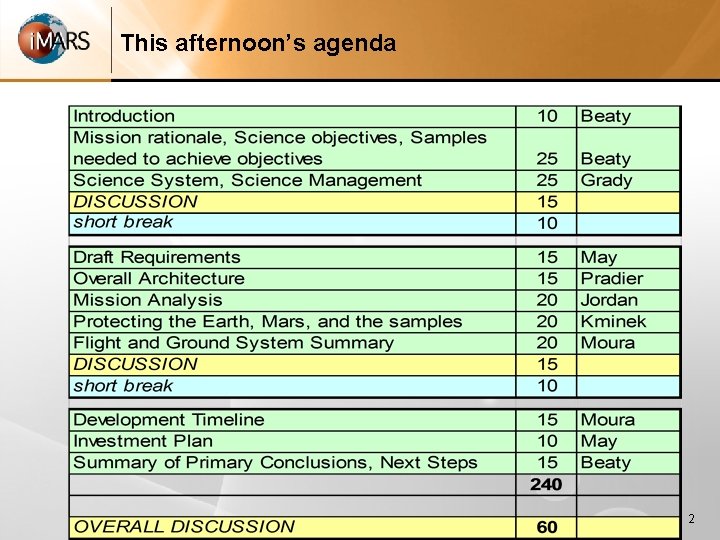
This afternoon’s agenda 2
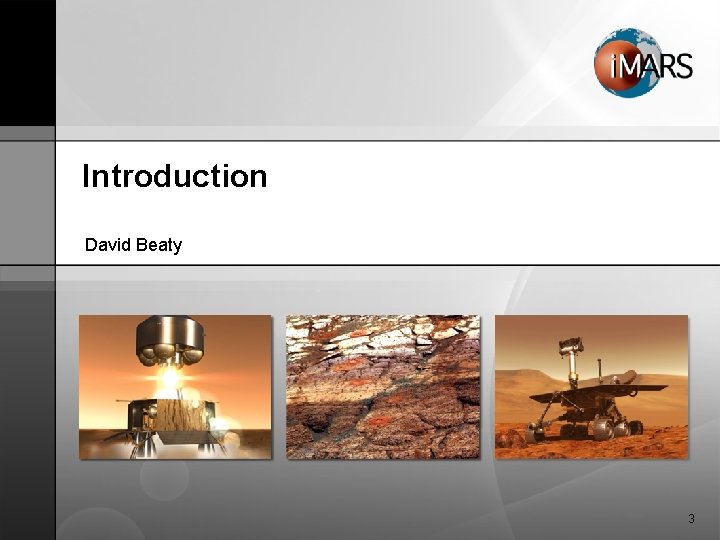
Introduction David Beaty 3
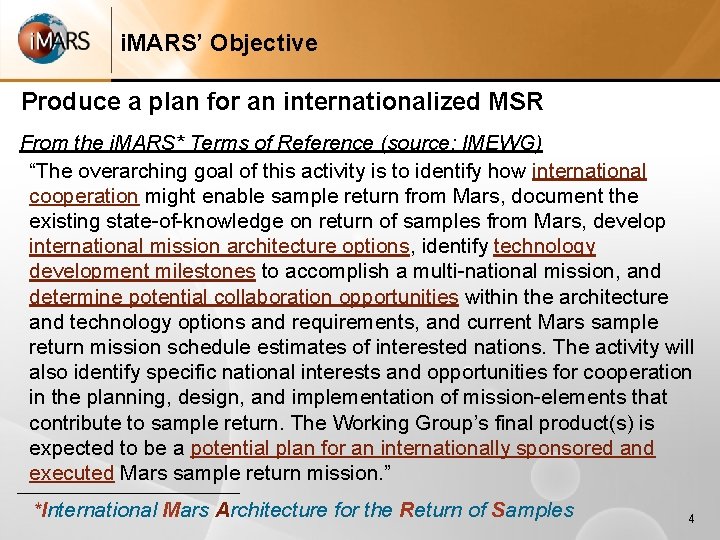
i. MARS’ Objective Produce a plan for an internationalized MSR From the i. MARS* Terms of Reference (source: IMEWG) “The overarching goal of this activity is to identify how international cooperation might enable sample return from Mars, document the existing state-of-knowledge on return of samples from Mars, develop international mission architecture options, identify technology development milestones to accomplish a multi-national mission, and determine potential collaboration opportunities within the architecture and technology options and requirements, and current Mars sample return mission schedule estimates of interested nations. The activity will also identify specific national interests and opportunities for cooperation in the planning, design, and implementation of mission-elements that contribute to sample return. The Working Group’s final product(s) is expected to be a potential plan for an internationally sponsored and executed Mars sample return mission. ” *International Mars Architecture for the Return of Samples 4
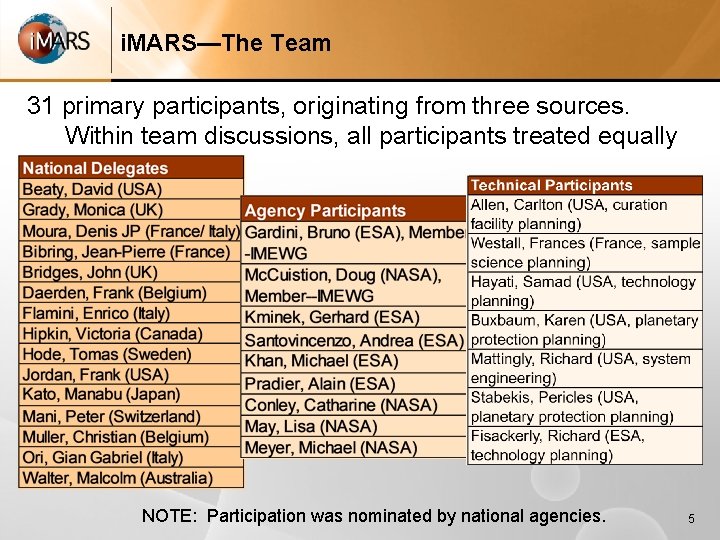
i. MARS—The Team 31 primary participants, originating from three sources. Within team discussions, all participants treated equally NOTE: Participation was nominated by national agencies. 5
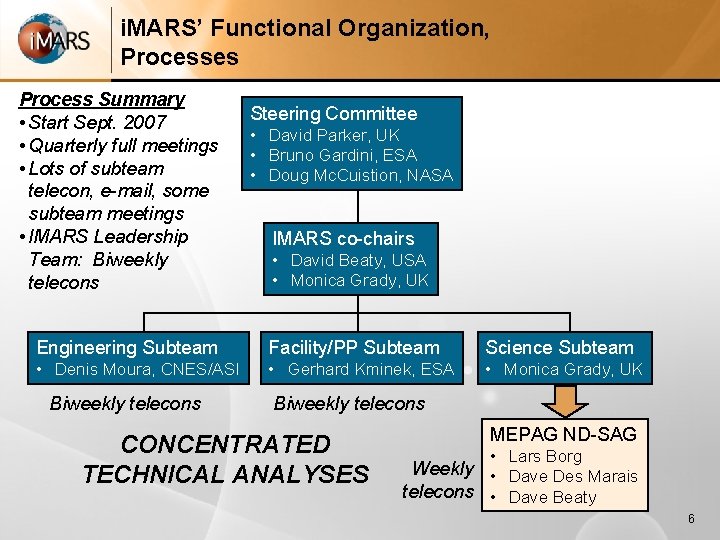
i. MARS’ Functional Organization, Processes Process Summary • Start Sept. 2007 • Quarterly full meetings • Lots of subteam telecon, e-mail, some subteam meetings • IMARS Leadership Team: Biweekly telecons Steering Committee • David Parker, UK • Bruno Gardini, ESA • Doug Mc. Cuistion, NASA IMARS co-chairs • David Beaty, USA • Monica Grady, UK Engineering Subteam Facility/PP Subteam Science Subteam • Denis Moura, CNES/ASI • Gerhard Kminek, ESA • Monica Grady, UK Biweekly telecons CONCENTRATED TECHNICAL ANALYSES MEPAG ND-SAG • Lars Borg Weekly • Dave Des Marais telecons • Dave Beaty 6
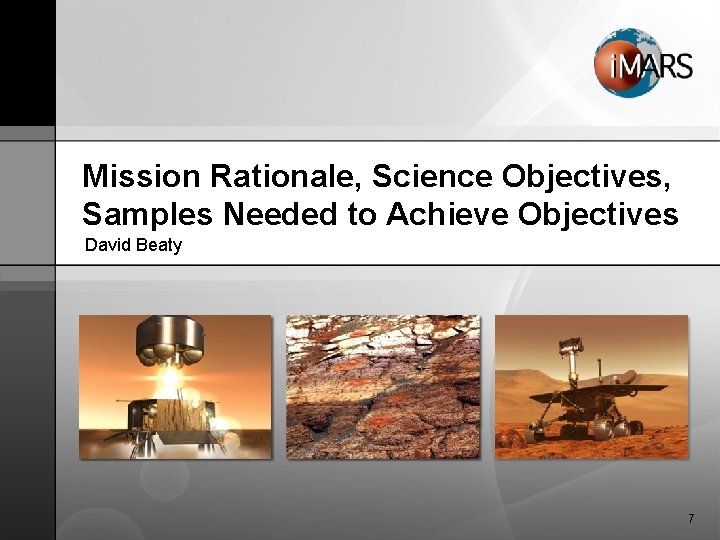
Mission Rationale, Science Objectives, Samples Needed to Achieve Objectives David Beaty 7
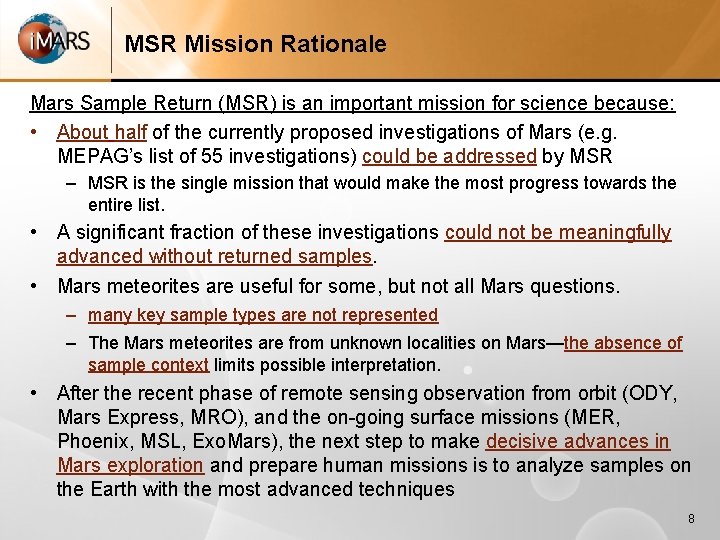
MSR Mission Rationale Mars Sample Return (MSR) is an important mission for science because: • About half of the currently proposed investigations of Mars (e. g. MEPAG’s list of 55 investigations) could be addressed by MSR – MSR is the single mission that would make the most progress towards the entire list. • A significant fraction of these investigations could not be meaningfully advanced without returned samples. • Mars meteorites are useful for some, but not all Mars questions. – many key sample types are not represented – The Mars meteorites are from unknown localities on Mars—the absence of sample context limits possible interpretation. • After the recent phase of remote sensing observation from orbit (ODY, Mars Express, MRO), and the on-going surface missions (MER, Phoenix, MSL, Exo. Mars), the next step to make decisive advances in Mars exploration and prepare human missions is to analyze samples on the Earth with the most advanced techniques 8
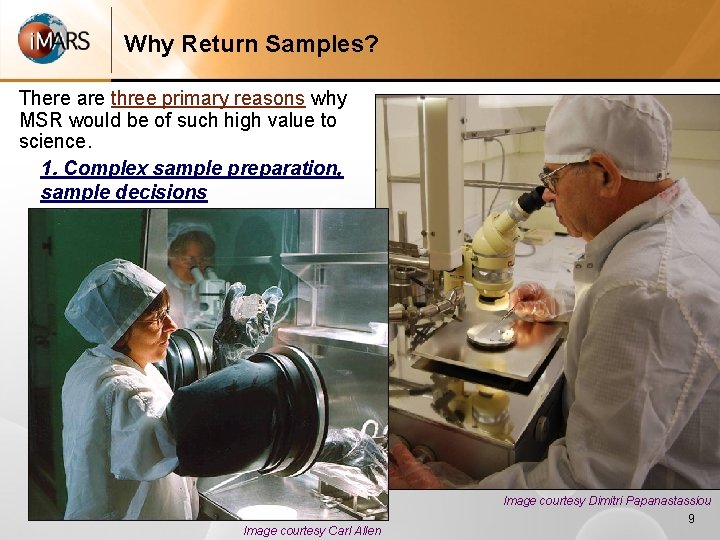
Why Return Samples? There are three primary reasons why MSR would be of such high value to science. 1. Complex sample preparation, sample decisions Image courtesy Carl Allen Image courtesy Dimitri Papanastassiou 9
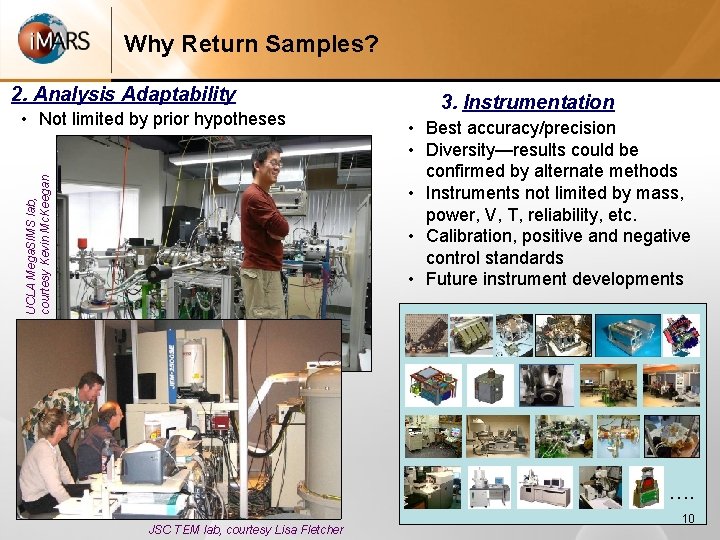
Why Return Samples? 2. Analysis Adaptability UCLA Mega. SIMS lab, courtesy Kevin Mc. Keegan • Not limited by prior hypotheses 3. Instrumentation • Best accuracy/precision • Diversity—results could be confirmed by alternate methods • Instruments not limited by mass, power, V, T, reliability, etc. • Calibration, positive and negative control standards • Future instrument developments …. JSC TEM lab, courtesy Lisa Fletcher 10
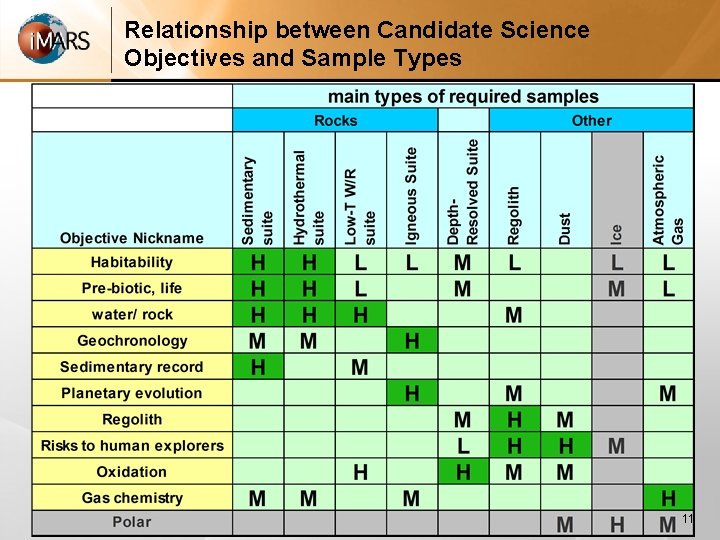
Relationship between Candidate Science Objectives and Sample Types 11
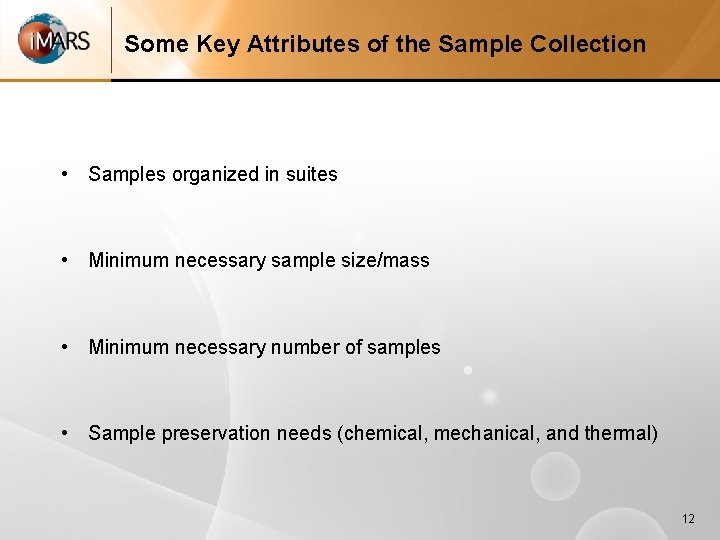
Some Key Attributes of the Sample Collection • Samples organized in suites • Minimum necessary sample size/mass • Minimum necessary number of samples • Sample preservation needs (chemical, mechanical, and thermal) 12
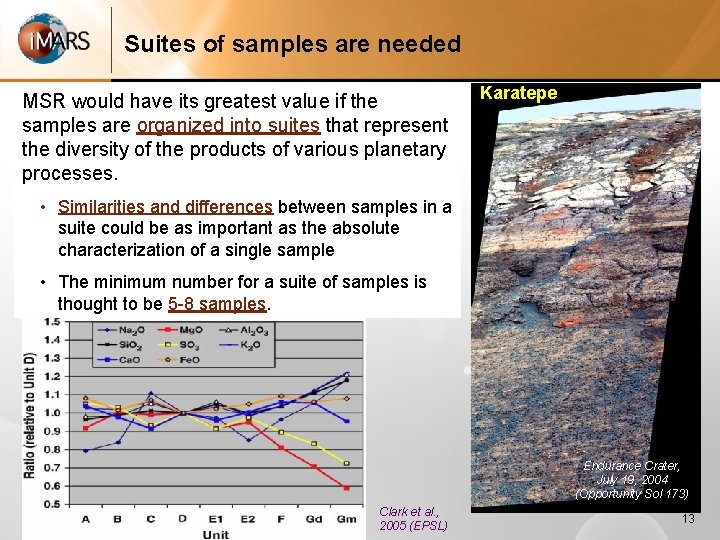
Suites of samples are needed MSR would have its greatest value if the samples are organized into suites that represent the diversity of the products of various planetary processes. Karatepe • Similarities and differences between samples in a suite could be as important as the absolute characterization of a single sample • The minimum number for a suite of samples is thought to be 5 -8 samples. Endurance Crater, July 19, 2004 (Opportunity Sol 173) Clark et al. , 2005 (EPSL) 13
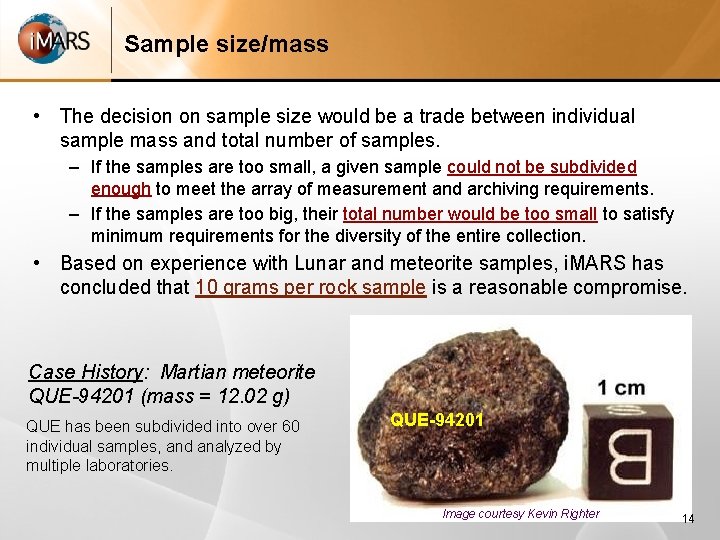
Sample size/mass • The decision on sample size would be a trade between individual sample mass and total number of samples. – If the samples are too small, a given sample could not be subdivided enough to meet the array of measurement and archiving requirements. – If the samples are too big, their total number would be too small to satisfy minimum requirements for the diversity of the entire collection. • Based on experience with Lunar and meteorite samples, i. MARS has concluded that 10 grams per rock sample is a reasonable compromise. Case History: Martian meteorite QUE-94201 (mass = 12. 02 g) QUE has been subdivided into over 60 individual samples, and analyzed by multiple laboratories. QUE-94201 Image courtesy Kevin Righter 14
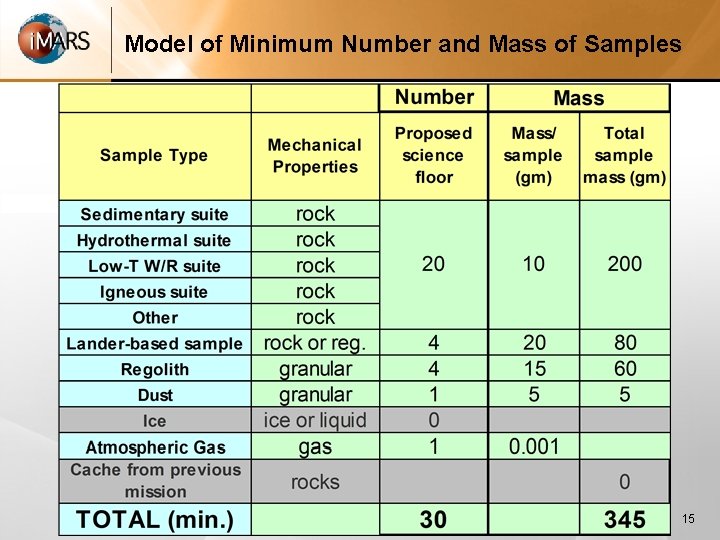
Model of Minimum Number and Mass of Samples 15
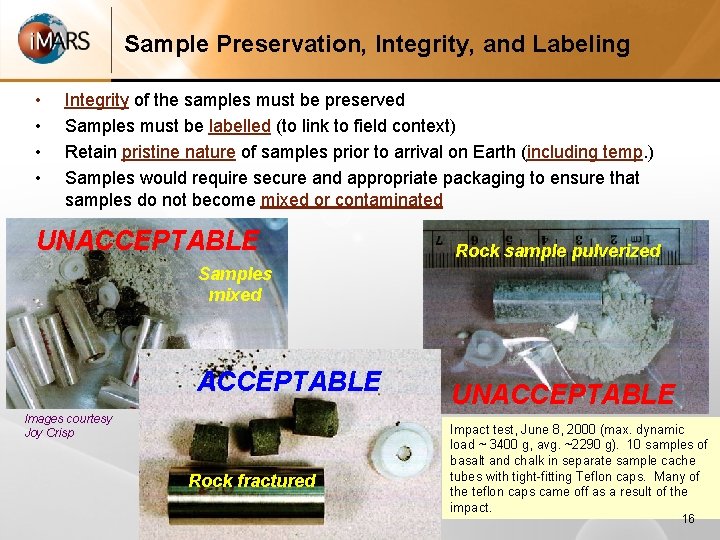
Sample Preservation, Integrity, and Labeling • • Integrity of the samples must be preserved Samples must be labelled (to link to field context) Retain pristine nature of samples prior to arrival on Earth (including temp. ) Samples would require secure and appropriate packaging to ensure that samples do not become mixed or contaminated UNACCEPTABLE Rock sample pulverized Samples mixed ACCEPTABLE Images courtesy Joy Crisp Rock fractured UNACCEPTABLE Impact test, June 8, 2000 (max. dynamic load ~ 3400 g, avg. ~2290 g). 10 samples of basalt and chalk in separate sample cache tubes with tight-fitting Teflon caps. Many of the teflon caps came off as a result of the impact. 16
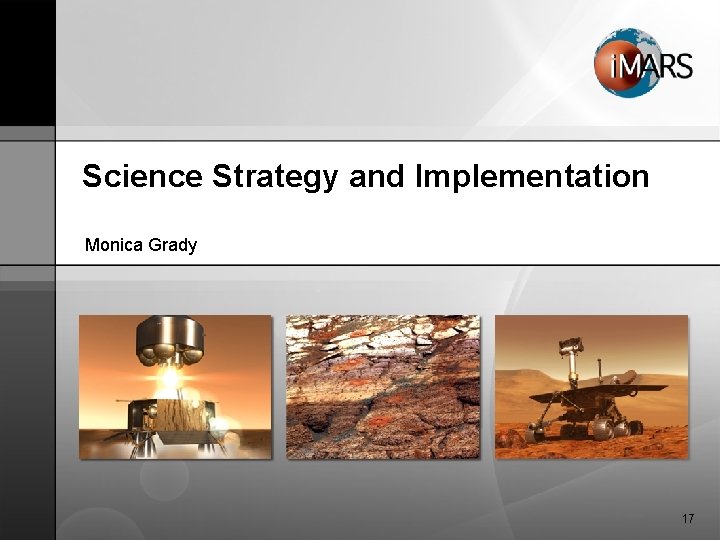
Science Strategy and Implementation Monica Grady 17
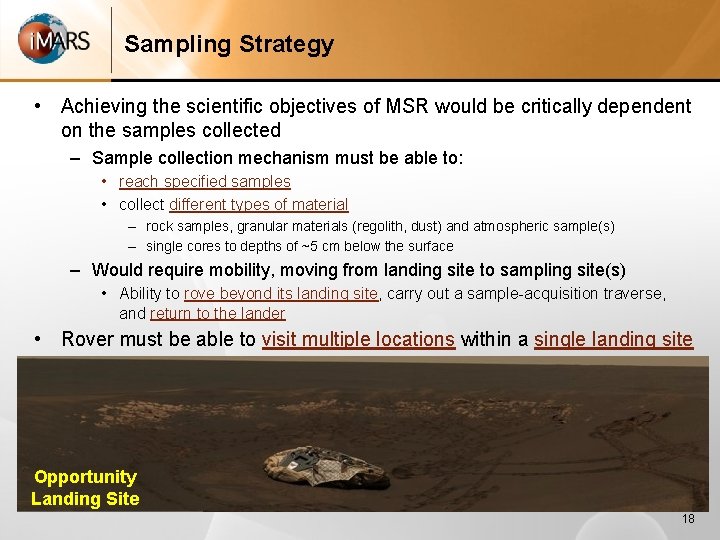
Sampling Strategy • Achieving the scientific objectives of MSR would be critically dependent on the samples collected – Sample collection mechanism must be able to: • reach specified samples • collect different types of material – rock samples, granular materials (regolith, dust) and atmospheric sample(s) – single cores to depths of ~5 cm below the surface – Would require mobility, moving from landing site to sampling site(s) • Ability to rove beyond its landing site, carry out a sample-acquisition traverse, and return to the lander • Rover must be able to visit multiple locations within a single landing site Opportunity Landing Site 18
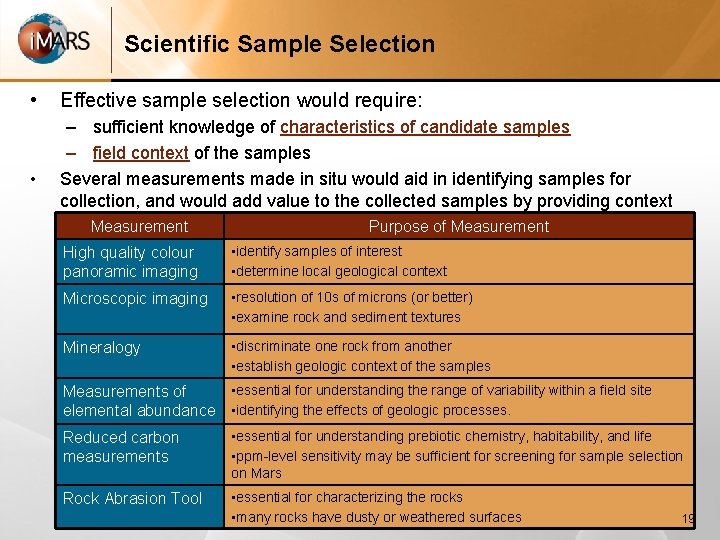
Scientific Sample Selection • • Effective sample selection would require: – sufficient knowledge of characteristics of candidate samples – field context of the samples Several measurements made in situ would aid in identifying samples for collection, and would add value to the collected samples by providing context Measurement Purpose of Measurement High quality colour panoramic imaging • identify samples of interest • determine local geological context Microscopic imaging • resolution of 10 s of microns (or better) • examine rock and sediment textures Mineralogy • discriminate one rock from another • establish geologic context of the samples • essential for understanding the range of variability within a field site Measurements of elemental abundance • identifying the effects of geologic processes. Reduced carbon measurements • essential for understanding prebiotic chemistry, habitability, and life • ppm-level sensitivity may be sufficient for screening for sample selection on Mars Rock Abrasion Tool • essential for characterizing the rocks • many rocks have dusty or weathered surfaces 19
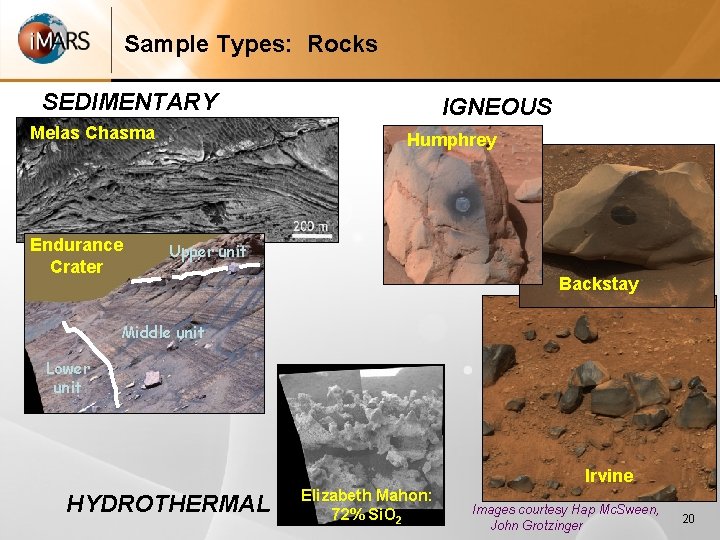
Sample Types: Rocks SEDIMENTARY Melas Chasma Endurance Crater IGNEOUS Humphrey Upper unit Backstay Middle unit Lower unit Irvine HYDROTHERMAL Elizabeth Mahon: 72% Si. O 2 Images courtesy Hap Mc. Sween, John Grotzinger 20
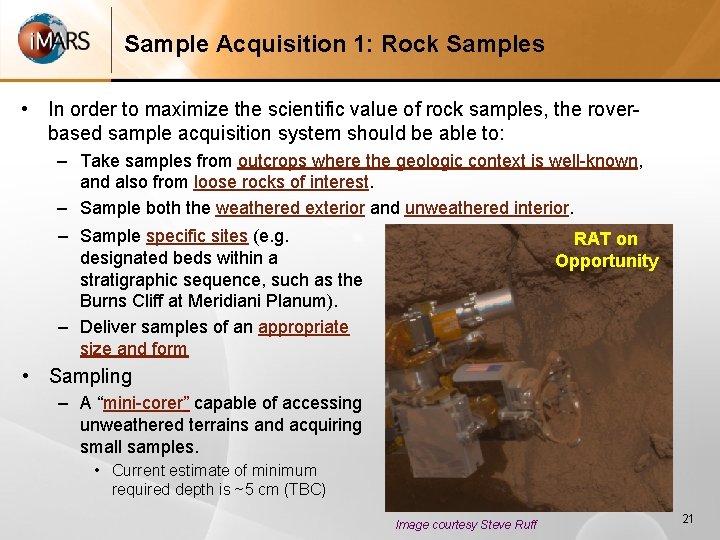
Sample Acquisition 1: Rock Samples • In order to maximize the scientific value of rock samples, the roverbased sample acquisition system should be able to: – Take samples from outcrops where the geologic context is well-known, and also from loose rocks of interest. – Sample both the weathered exterior and unweathered interior. – Sample specific sites (e. g. designated beds within a stratigraphic sequence, such as the Burns Cliff at Meridiani Planum). – Deliver samples of an appropriate size and form RAT on Opportunity • Sampling – A “mini-corer” capable of accessing unweathered terrains and acquiring small samples. • Current estimate of minimum required depth is ~5 cm (TBC) Image courtesy Steve Ruff 21
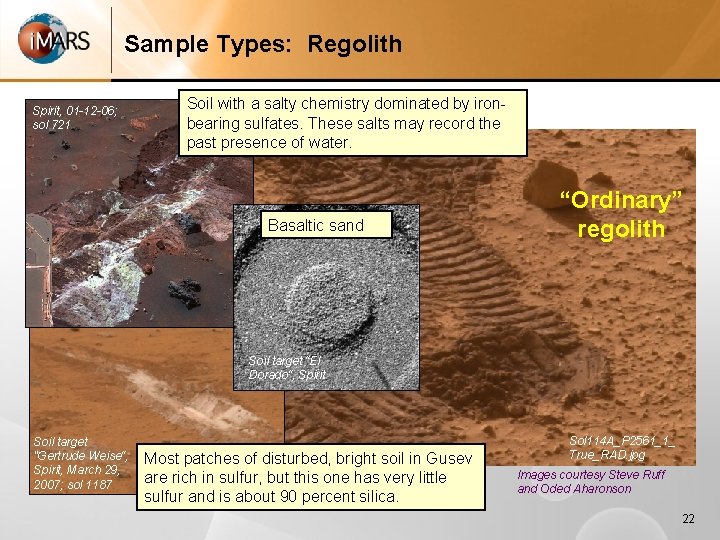
Sample Types: Regolith Spirit, 01 -12 -06; sol 721 Soil with a salty chemistry dominated by ironbearing sulfates. These salts may record the past presence of water. Basaltic sand “Ordinary” regolith Soil target “El Dorado“, Spirit Soil target "Gertrude Weise“, Spirit, March 29, 2007; sol 1187 Most patches of disturbed, bright soil in Gusev are rich in sulfur, but this one has very little sulfur and is about 90 percent silica. Sol 114 A_P 2561_1_ True_RAD. jpg Images courtesy Steve Ruff and Oded Aharonson 22
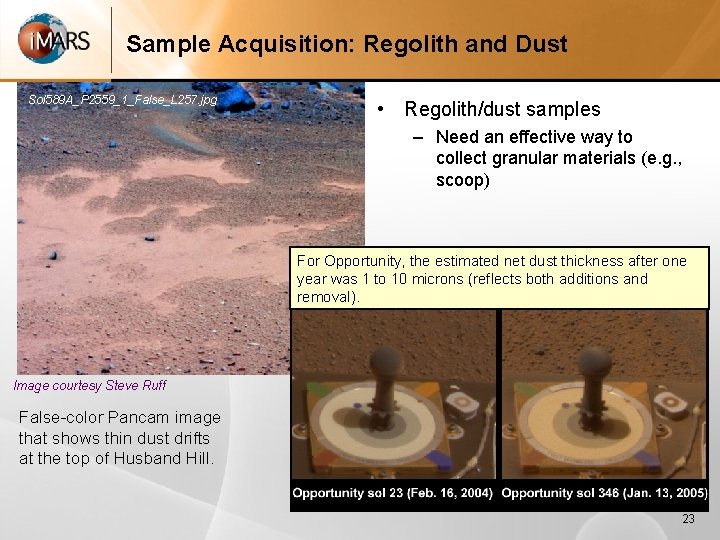
Sample Acquisition: Regolith and Dust Sol 589 A_P 2559_1_False_L 257. jpg • Regolith/dust samples – Need an effective way to collect granular materials (e. g. , scoop) For Opportunity, the estimated net dust thickness after one year was 1 to 10 microns (reflects both additions and removal). Image courtesy Steve Ruff False-color Pancam image that shows thin dust drifts at the top of Husband Hill. 23
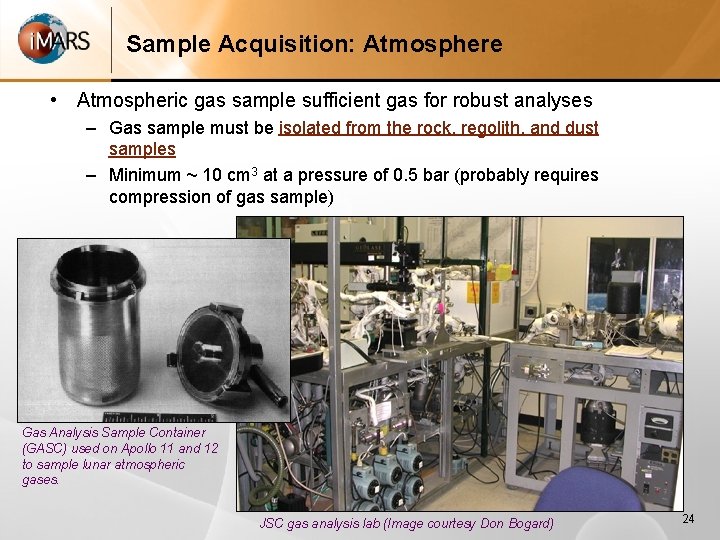
Sample Acquisition: Atmosphere • Atmospheric gas sample sufficient gas for robust analyses – Gas sample must be isolated from the rock, regolith, and dust samples – Minimum ~ 10 cm 3 at a pressure of 0. 5 bar (probably requires compression of gas sample) Gas Analysis Sample Container (GASC) used on Apollo 11 and 12 to sample lunar atmospheric gases. JSC gas analysis lab (Image courtesy Don Bogard) 24
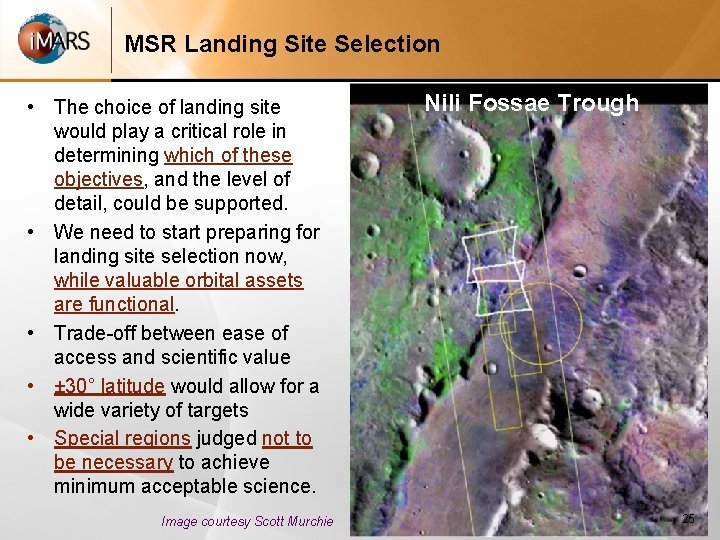
MSR Landing Site Selection • The choice of landing site would play a critical role in determining which of these objectives, and the level of detail, could be supported. • We need to start preparing for landing site selection now, while valuable orbital assets are functional. • Trade-off between ease of access and scientific value • ± 30° latitude would allow for a wide variety of targets • Special regions judged not to be necessary to achieve minimum acceptable science. Image courtesy Scott Murchie Nili Fossae Trough 25
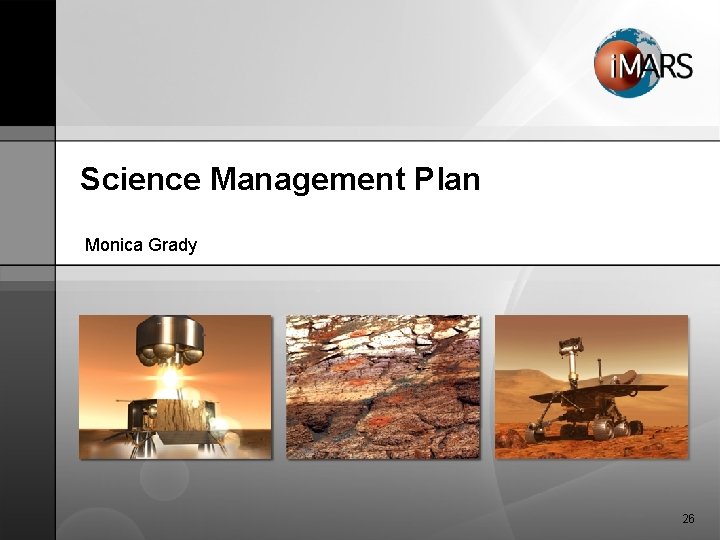
Science Management Plan Monica Grady 26
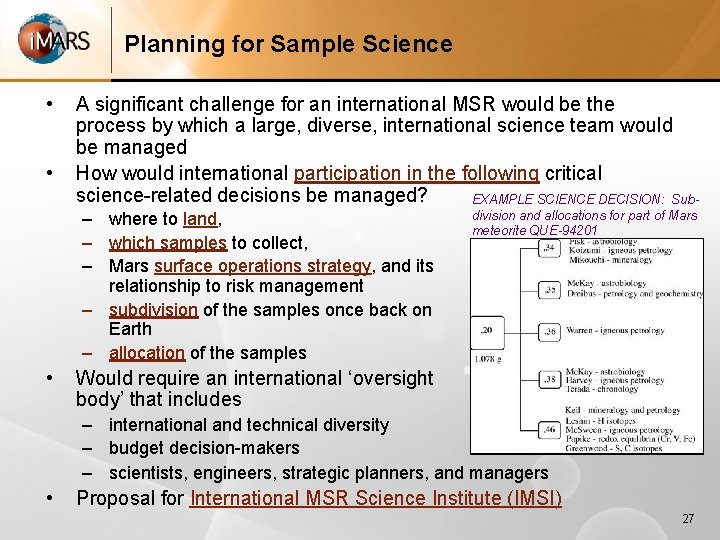
Planning for Sample Science • • A significant challenge for an international MSR would be the process by which a large, diverse, international science team would be managed How would international participation in the following critical science-related decisions be managed? EXAMPLE SCIENCE DECISION: Sub– where to land, – which samples to collect, – Mars surface operations strategy, and its relationship to risk management – subdivision of the samples once back on Earth – allocation of the samples • division and allocations for part of Mars meteorite QUE-94201 Would require an international ‘oversight body’ that includes – international and technical diversity – budget decision-makers – scientists, engineers, strategic planners, and managers • Proposal for International MSR Science Institute (IMSI) 27
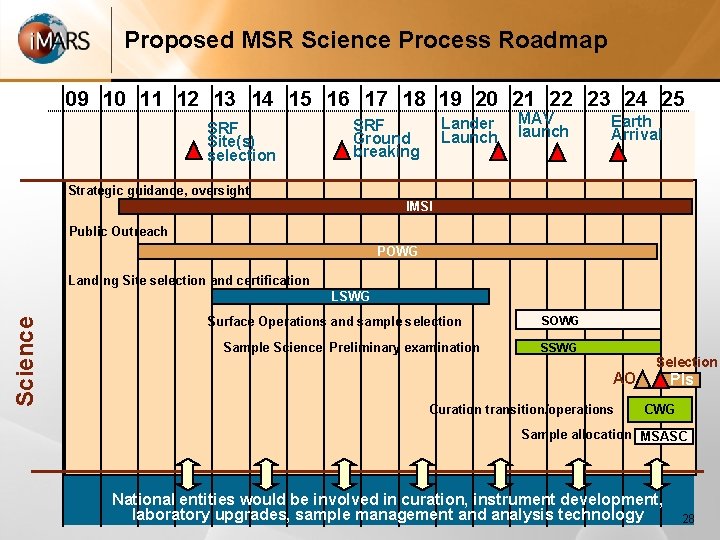
Proposed MSR Science Process Roadmap 09 10 11 12 13 14 15 16 17 18 19 20 21 22 23 24 25 SRF Site(s) selection Lander Launch SRF Ground breaking MAV launch Earth Arrival Strategic guidance, oversight IMSI Public Outreach POWG Landing Site selection and certification Science LSWG Surface Operations and sample selection Sample Science, Preliminary examination SOWG SSWG AO Curation transition/operations Selection PIs CWG Sample allocation MSASC National entities would be involved in curation, instrument development, laboratory upgrades, sample management and analysis technology 28
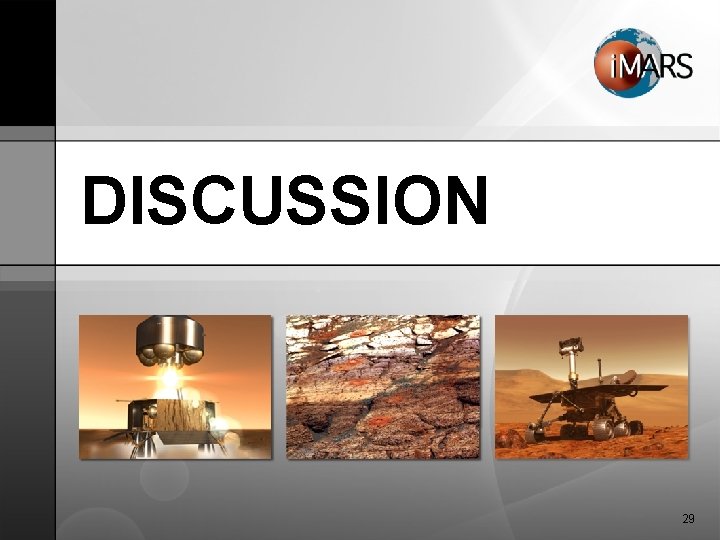
DISCUSSION 29
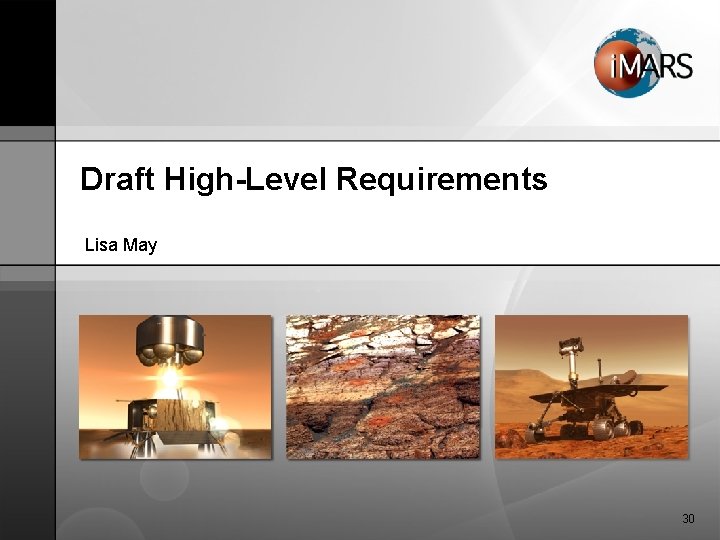
Draft High-Level Requirements Lisa May 30
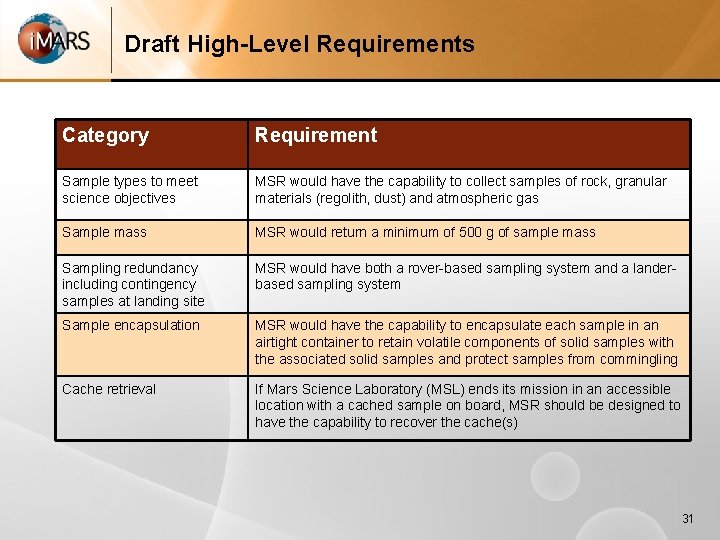
Draft High-Level Requirements Category Requirement Sample types to meet science objectives MSR would have the capability to collect samples of rock, granular materials (regolith, dust) and atmospheric gas Sample mass MSR would return a minimum of 500 g of sample mass Sampling redundancy including contingency samples at landing site MSR would have both a rover-based sampling system and a landerbased sampling system Sample encapsulation MSR would have the capability to encapsulate each sample in an airtight container to retain volatile components of solid samples with the associated solid samples and protect samples from commingling Cache retrieval If Mars Science Laboratory (MSL) ends its mission in an accessible location with a cached sample on board, MSR should be designed to have the capability to recover the cache(s) 31
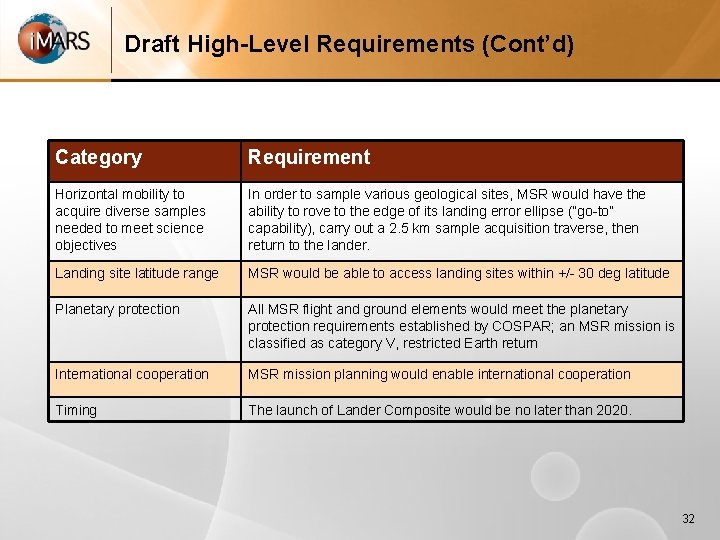
Draft High-Level Requirements (Cont’d) Category Requirement Horizontal mobility to acquire diverse samples needed to meet science objectives In order to sample various geological sites, MSR would have the ability to rove to the edge of its landing error ellipse (“go-to” capability), carry out a 2. 5 km sample acquisition traverse, then return to the lander. Landing site latitude range MSR would be able to access landing sites within +/- 30 deg latitude Planetary protection All MSR flight and ground elements would meet the planetary protection requirements established by COSPAR; an MSR mission is classified as category V, restricted Earth return International cooperation MSR mission planning would enable international cooperation Timing The launch of Lander Composite would be no later than 2020. 32
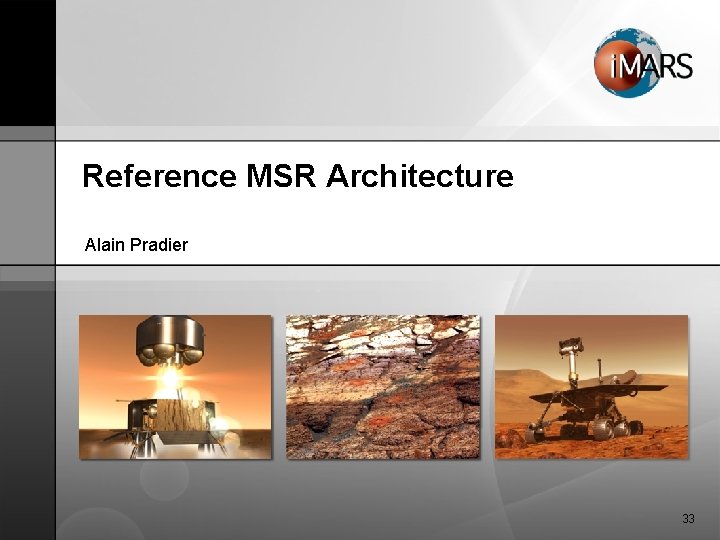
Reference MSR Architecture Alain Pradier 33
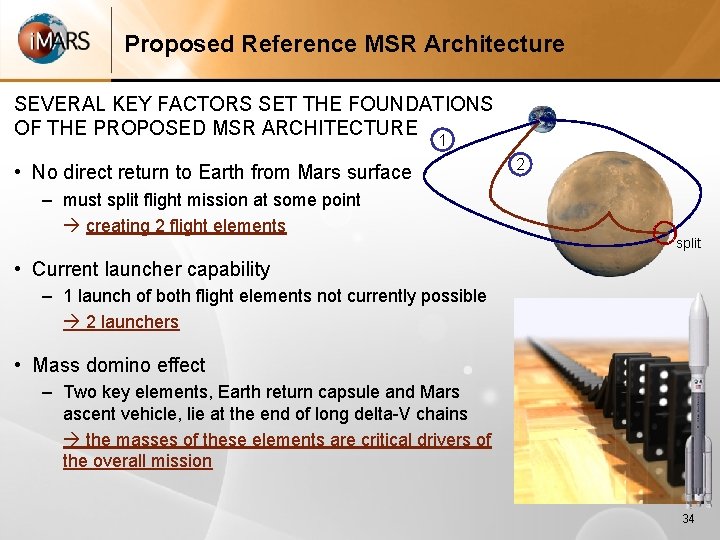
Proposed Reference MSR Architecture SEVERAL KEY FACTORS SET THE FOUNDATIONS OF THE PROPOSED MSR ARCHITECTURE 1 • No direct return to Earth from Mars surface – must split flight mission at some point creating 2 flight elements 2 split • Current launcher capability – 1 launch of both flight elements not currently possible 2 launchers • Mass domino effect – Two key elements, Earth return capsule and Mars ascent vehicle, lie at the end of long delta-V chains the masses of these elements are critical drivers of the overall mission 34
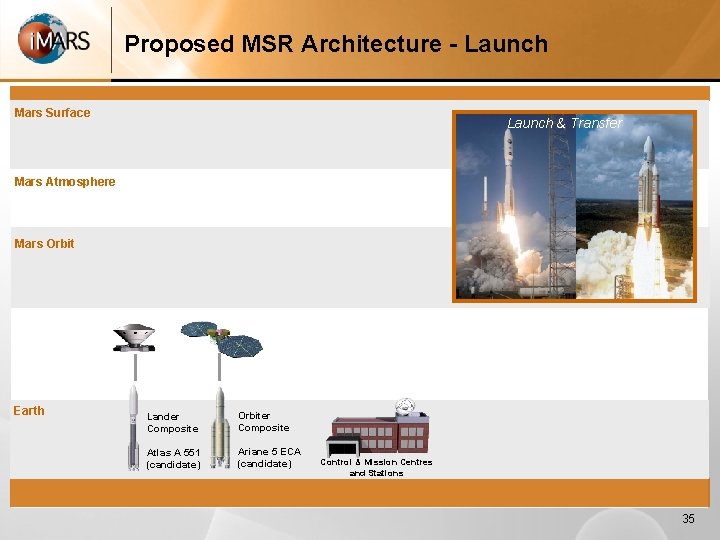
Proposed MSR Architecture - Launch Mars Surface Launch & Transfer Mars Atmosphere Mars Orbit Earth Lander Composite Orbiter Composite Atlas A 551 (candidate) Ariane 5 ECA (candidate) Control & Mission Centres and Stations 35
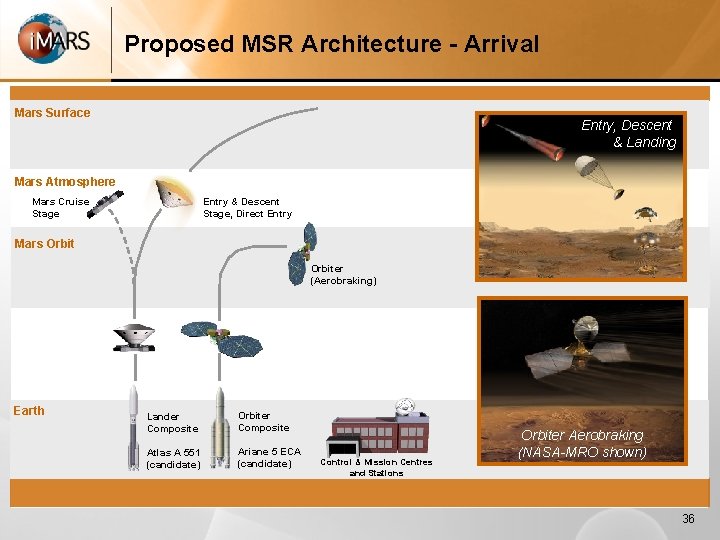
Proposed MSR Architecture - Arrival Mars Surface Entry, Descent & Landing Mars Atmosphere Entry & Descent Stage, Direct Entry Mars Cruise Stage Mars Orbiter (Aerobraking) Earth Lander Composite Orbiter Composite Atlas A 551 (candidate) Ariane 5 ECA (candidate) Control & Mission Centres and Stations Orbiter Aerobraking (NASA-MRO shown) 36
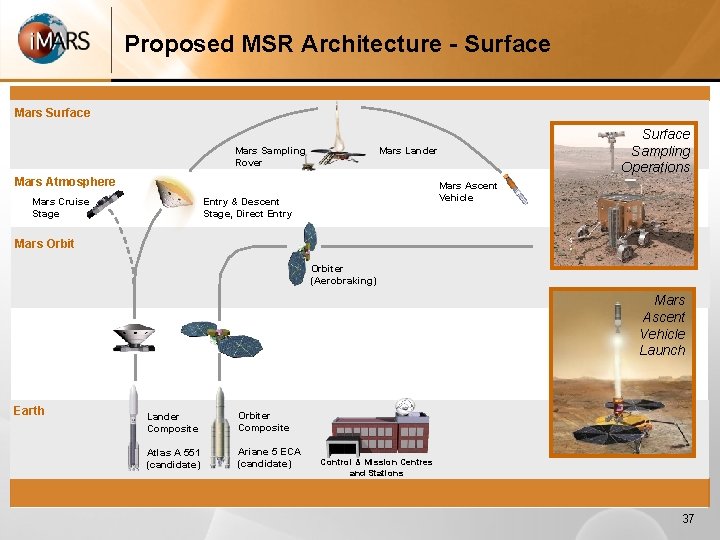
Proposed MSR Architecture - Surface Mars Sampling Rover Mars Lander Mars Atmosphere Mars Ascent Vehicle Entry & Descent Stage, Direct Entry Mars Cruise Stage Surface Sampling Operations Mars Orbiter (Aerobraking) Mars Ascent Vehicle Launch Earth Lander Composite Orbiter Composite Atlas A 551 (candidate) Ariane 5 ECA (candidate) Control & Mission Centres and Stations 37
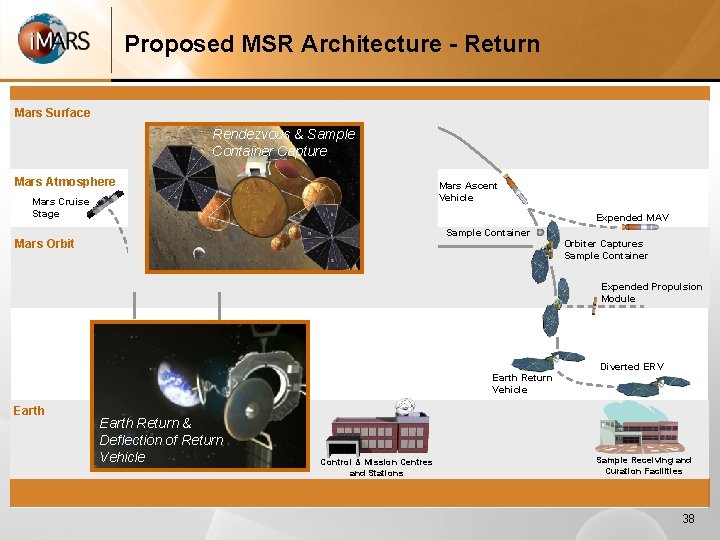
Proposed MSR Architecture - Return Mars Surface Rendezvous & Sample Mars Sampling Container Capture Mars Lander Rover Mars Atmosphere Mars Ascent Vehicle Entry & Descent Stage, Direct Entry Mars Cruise Stage Expended MAV Sample Container Mars Orbiter (Aerobraking) Expended Propulsion Module Earth Return Vehicle Earth Lander Earth Return & Composite Deflection of Return Vehicle Atlas A 551 Orbiter Captures Sample Container Diverted ERV Orbiter Composite Ariane 5 ECA Control & Mission Centres and Stations Sample Receiving and Curation Facilities 38
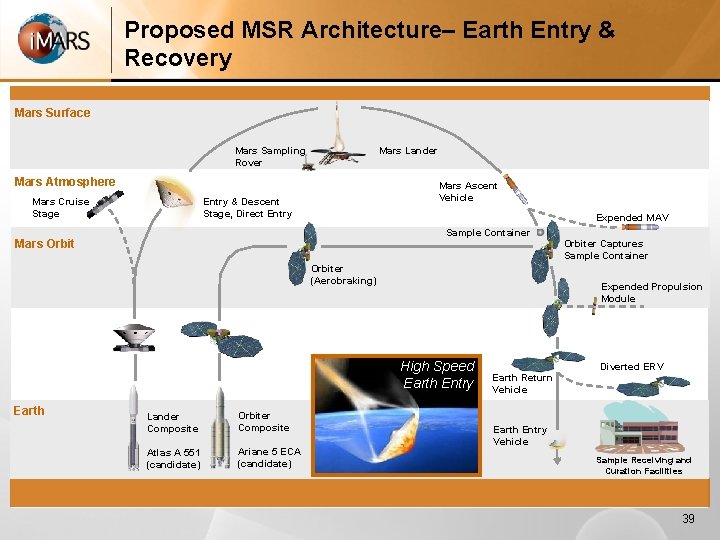
Proposed MSR Architecture– Earth Entry & Recovery Mars Surface Mars Sampling Rover Mars Lander Mars Atmosphere Mars Ascent Vehicle Entry & Descent Stage, Direct Entry Mars Cruise Stage Expended MAV Sample Container Mars Orbiter (Aerobraking) Expended Propulsion Module High Speed Earth Entry Earth Lander Composite Orbiter Composite Atlas A 551 (candidate) Ariane 5 ECA (candidate) Orbiter Captures Sample Container Earth Return Vehicle Diverted ERV Earth Entry Vehicle Control & Mission Centres and Stations Sample Receiving and Curation Facilities 39
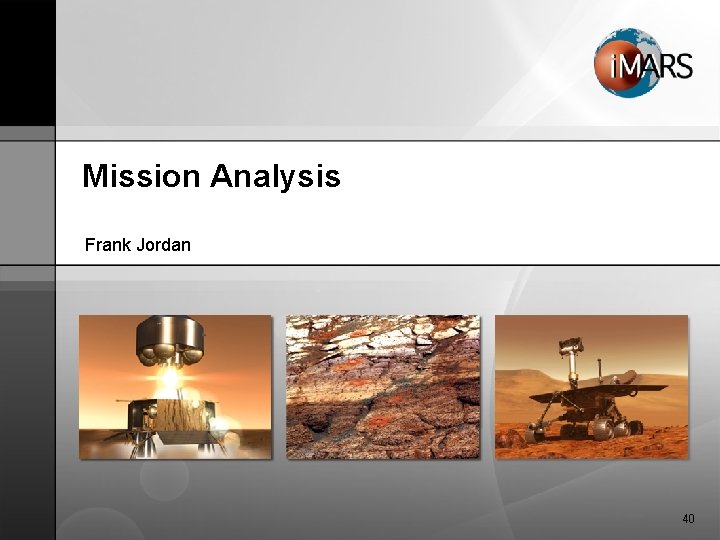
Mission Analysis Frank Jordan 40
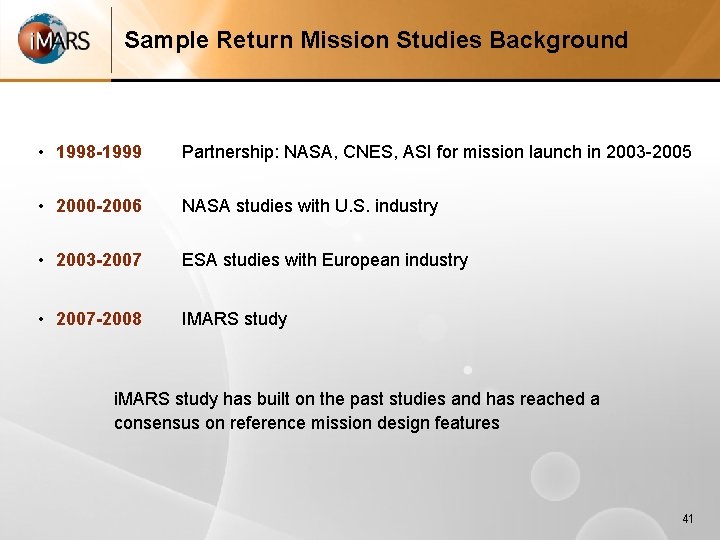
Sample Return Mission Studies Background • 1998 -1999 Partnership: NASA, CNES, ASI for mission launch in 2003 -2005 • 2000 -2006 NASA studies with U. S. industry • 2003 -2007 ESA studies with European industry • 2007 -2008 IMARS study i. MARS study has built on the past studies and has reached a consensus on reference mission design features 41
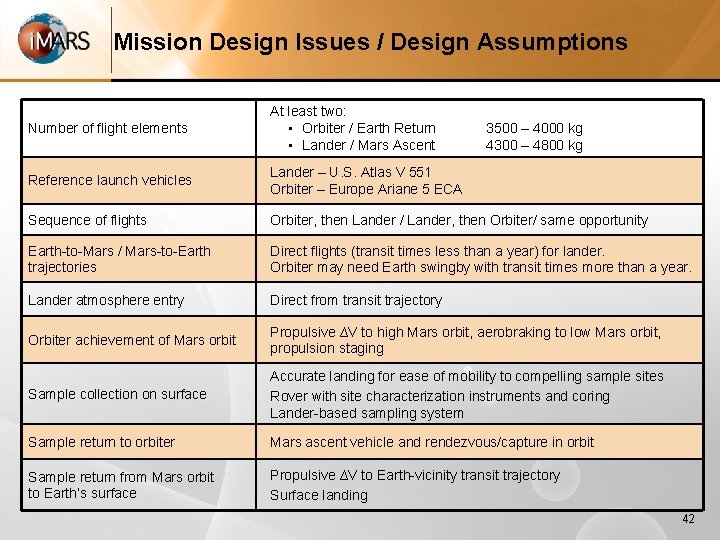
Mission Design Issues / Design Assumptions Number of flight elements At least two: • Orbiter / Earth Return • Lander / Mars Ascent Reference launch vehicles Lander – U. S. Atlas V 551 Orbiter – Europe Ariane 5 ECA Sequence of flights Orbiter, then Lander / Lander, then Orbiter/ same opportunity Earth-to-Mars / Mars-to-Earth trajectories Direct flights (transit times less than a year) for lander. Orbiter may need Earth swingby with transit times more than a year. Lander atmosphere entry Direct from transit trajectory Orbiter achievement of Mars orbit Propulsive ∆V to high Mars orbit, aerobraking to low Mars orbit, propulsion staging Sample collection on surface Accurate landing for ease of mobility to compelling sample sites Rover with site characterization instruments and coring Lander-based sampling system Sample return to orbiter Mars ascent vehicle and rendezvous/capture in orbit Sample return from Mars orbit to Earth’s surface Propulsive ∆V to Earth-vicinity transit trajectory Surface landing 3500 – 4000 kg 4300 – 4800 kg 42
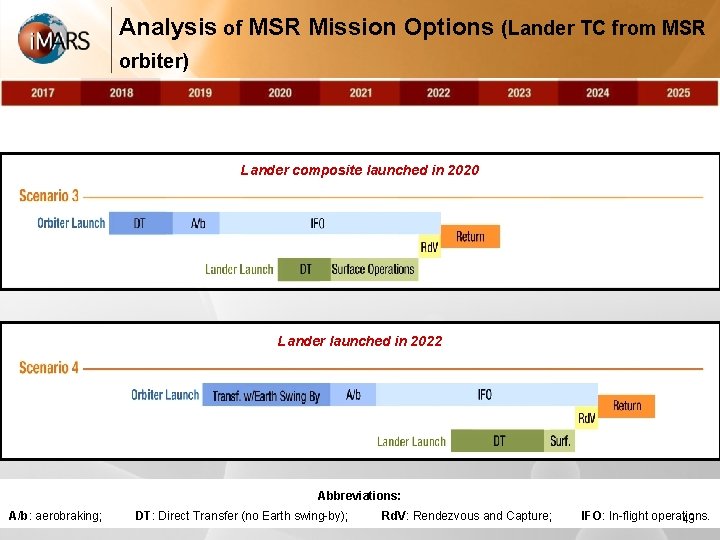
Analysis of MSR Mission Options (Lander TC from MSR orbiter) Lander composite launched in 2020 Lander launched in 2022 Abbreviations: A/b: aerobraking; DT: Direct Transfer (no Earth swing-by); Rd. V: Rendezvous and Capture; IFO: In-flight operations. 43
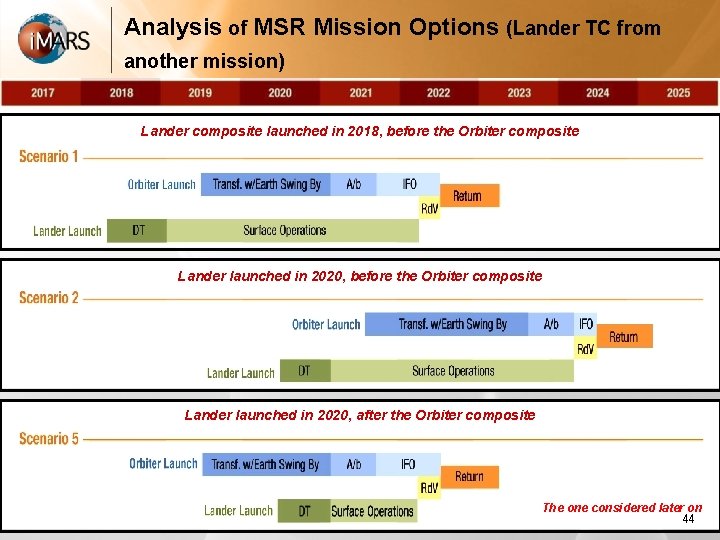
Analysis of MSR Mission Options (Lander TC from another mission) Lander composite launched in 2018, before the Orbiter composite Lander launched in 2020, after the Orbiter composite The one considered later on 44
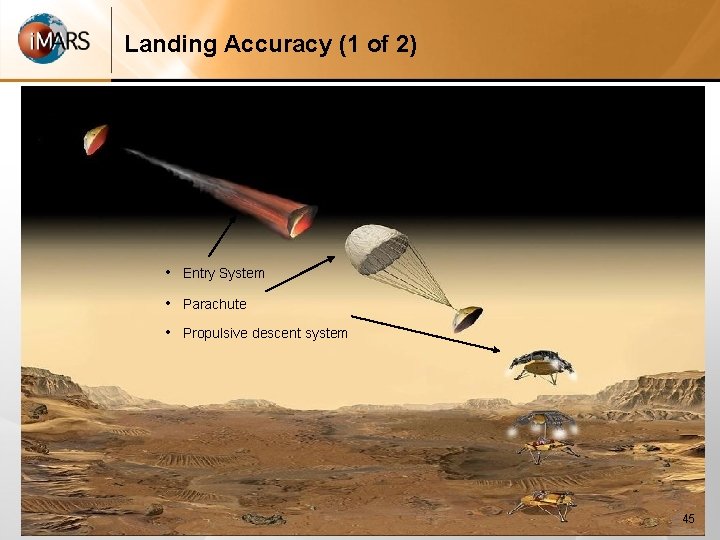
Landing Accuracy (1 of 2) • Entry System • Parachute • Propulsive descent system 45
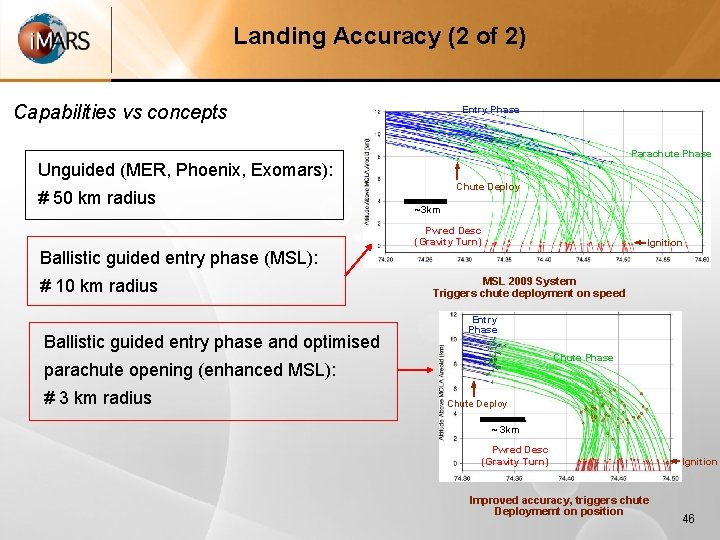
Landing Accuracy (2 of 2) Capabilities vs concepts Entry Phase Parachute Phase Unguided (MER, Phoenix, Exomars): # 50 km radius Chute Deploy ~3 km Pwred Desc (Gravity Turn) Ignition Ballistic guided entry phase (MSL): # 10 km radius Ballistic guided entry phase and optimised MSL 2009 System Triggers chute deployment on speed Entry Phase Chute Phase parachute opening (enhanced MSL): # 3 km radius Chute Deploy ~ 3 km Pwred Desc (Gravity Turn) Improved accuracy, triggers chute Deploymemt on position Ignition 46
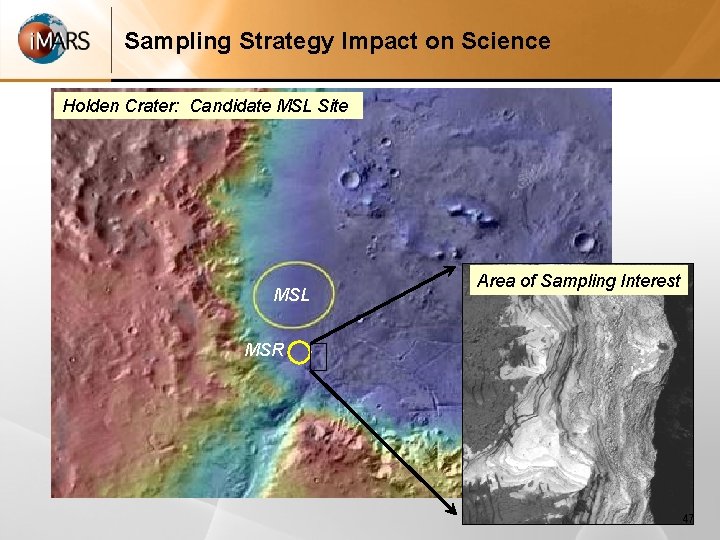
Sampling Strategy Impact on Science Holden Crater: Candidate MSL Site MSL Area of Sampling Interest MSR 47
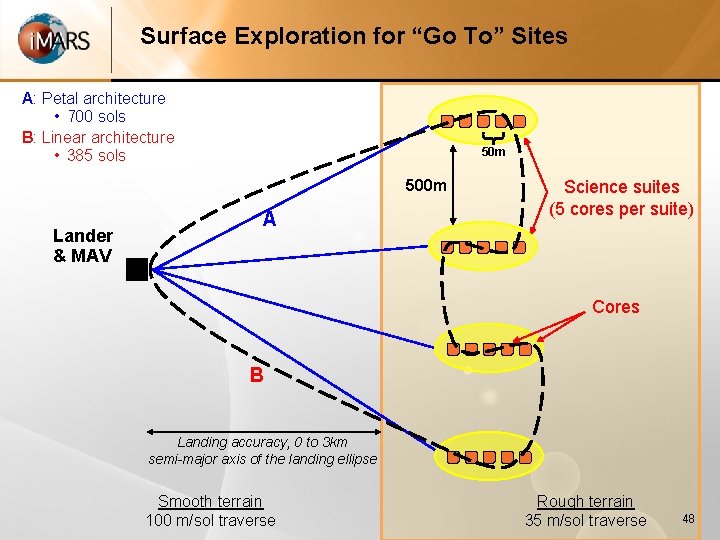
Surface Exploration for “Go To” Sites A: Petal architecture • 700 sols B: Linear architecture • 385 sols 50 m 500 m Lander & MAV A Science suites (5 cores per suite) Cores B Landing accuracy, 0 to 3 km semi-major axis of the landing ellipse Smooth terrain 100 m/sol traverse Rough terrain 35 m/sol traverse 48
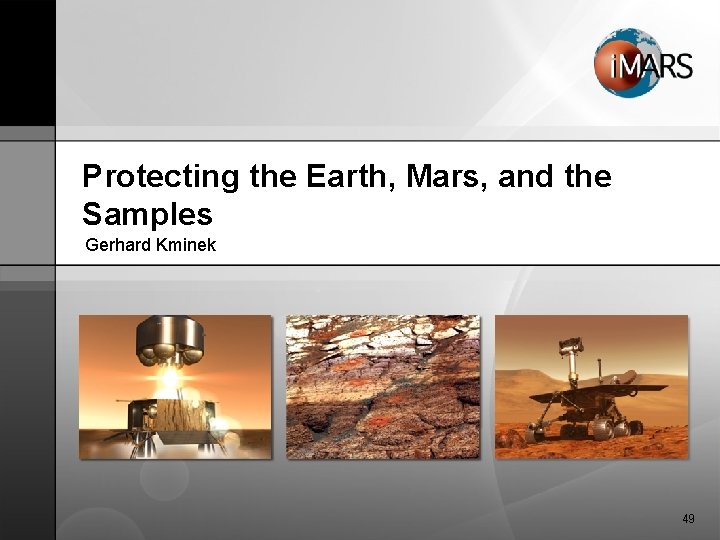
Protecting the Earth, Mars, and the Samples Gerhard Kminek 49
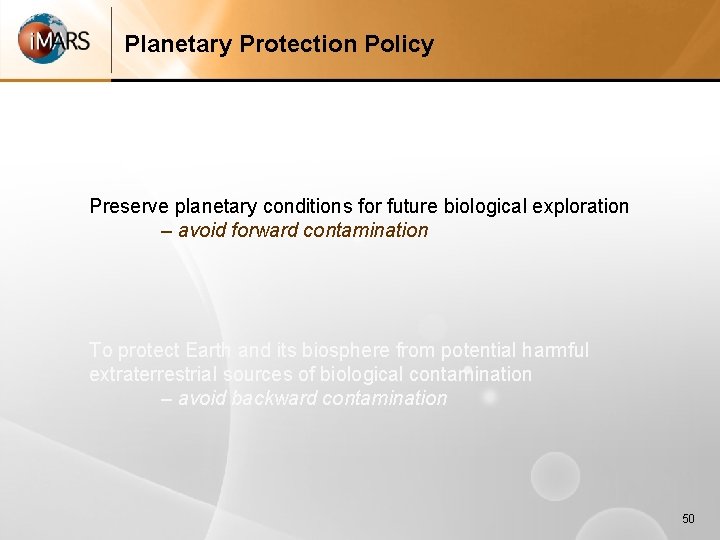
Planetary Protection Policy Preserve planetary conditions for future biological exploration – avoid forward contamination To protect Earth and its biosphere from potential harmful extraterrestrial sources of biological contamination – avoid backward contamination 50
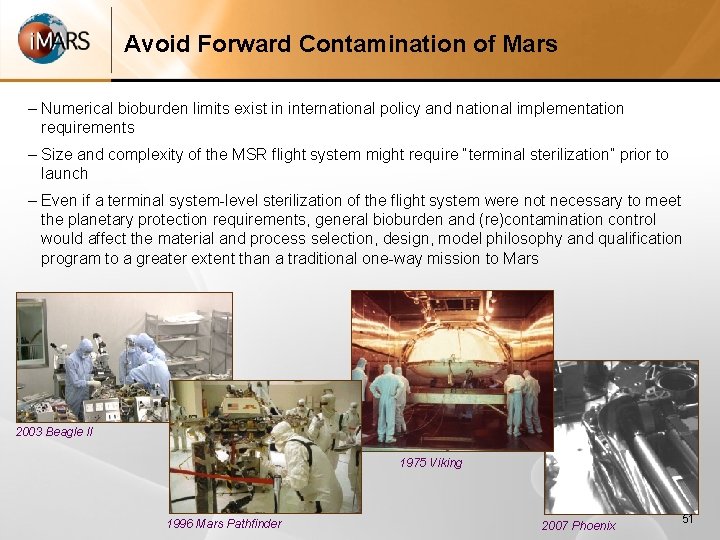
Avoid Forward Contamination of Mars – Numerical bioburden limits exist in international policy and national implementation requirements – Size and complexity of the MSR flight system might require “terminal sterilization” prior to launch – Even if a terminal system-level sterilization of the flight system were not necessary to meet the planetary protection requirements, general bioburden and (re)contamination control would affect the material and process selection, design, model philosophy and qualification program to a greater extent than a traditional one-way mission to Mars 2003 Beagle II 1975 Viking 1996 Mars Pathfinder 2007 Phoenix 51
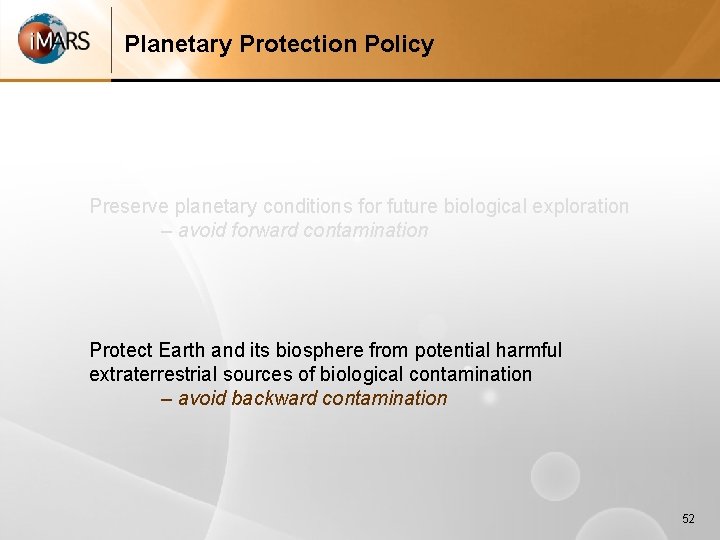
Planetary Protection Policy Preserve planetary conditions for future biological exploration – avoid forward contamination Protect Earth and its biosphere from potential harmful extraterrestrial sources of biological contamination – avoid backward contamination 52
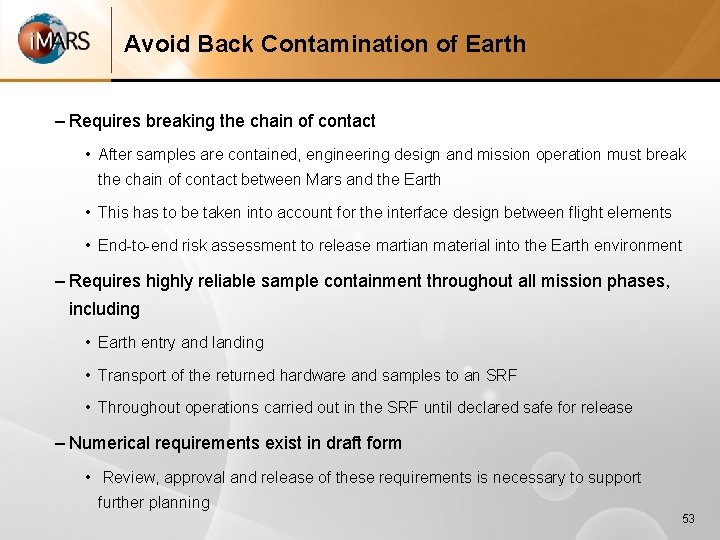
Avoid Back Contamination of Earth – Requires breaking the chain of contact • After samples are contained, engineering design and mission operation must break the chain of contact between Mars and the Earth • This has to be taken into account for the interface design between flight elements • End-to-end risk assessment to release martian material into the Earth environment – Requires highly reliable sample containment throughout all mission phases, including • Earth entry and landing • Transport of the returned hardware and samples to an SRF • Throughout operations carried out in the SRF until declared safe for release – Numerical requirements exist in draft form • Review, approval and release of these requirements is necessary to support further planning 53
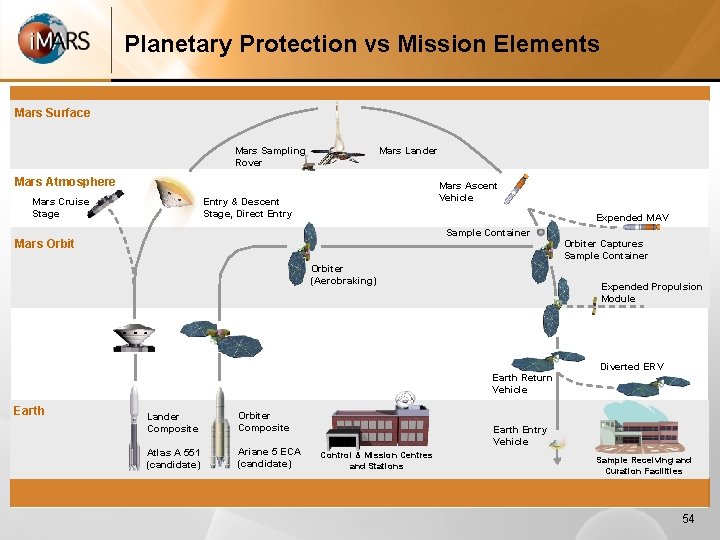
Planetary Protection vs Mission Elements Mars Surface Mars Sampling Rover Mars Lander Mars Atmosphere Mars Ascent Vehicle Entry & Descent Stage, Direct Entry Mars Cruise Stage Expended MAV Sample Container Mars Orbiter (Aerobraking) Expended Propulsion Module Earth Return Vehicle Earth Lander Composite Orbiter Composite Atlas A 551 (candidate) Ariane 5 ECA (candidate) Orbiter Captures Sample Container Diverted ERV Earth Entry Vehicle Control & Mission Centres and Stations Sample Receiving and Curation Facilities 54
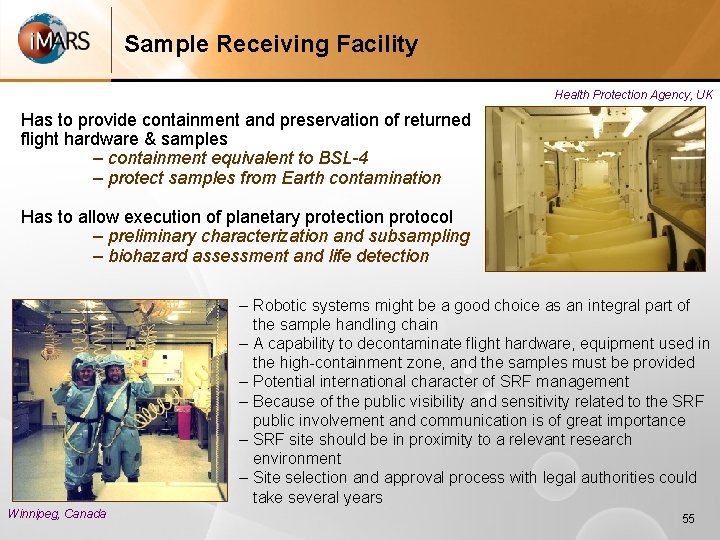
Sample Receiving Facility Health Protection Agency, UK Has to provide containment and preservation of returned flight hardware & samples – containment equivalent to BSL-4 – protect samples from Earth contamination Has to allow execution of planetary protection protocol – preliminary characterization and subsampling – biohazard assessment and life detection – Robotic systems might be a good choice as an integral part of the sample handling chain – A capability to decontaminate flight hardware, equipment used in the high-containment zone, and the samples must be provided – Potential international character of SRF management – Because of the public visibility and sensitivity related to the SRF public involvement and communication is of great importance – SRF site should be in proximity to a relevant research environment – Site selection and approval process with legal authorities could take several years Winnipeg, Canada 55
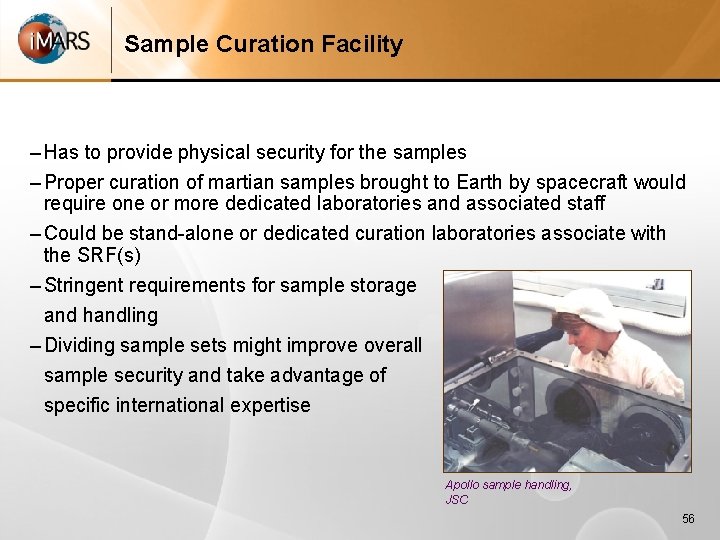
Sample Curation Facility – Has to provide physical security for the samples – Proper curation of martian samples brought to Earth by spacecraft would require one or more dedicated laboratories and associated staff – Could be stand-alone or dedicated curation laboratories associate with the SRF(s) – Stringent requirements for sample storage and handling – Dividing sample sets might improve overall sample security and take advantage of specific international expertise Apollo sample handling, JSC 56
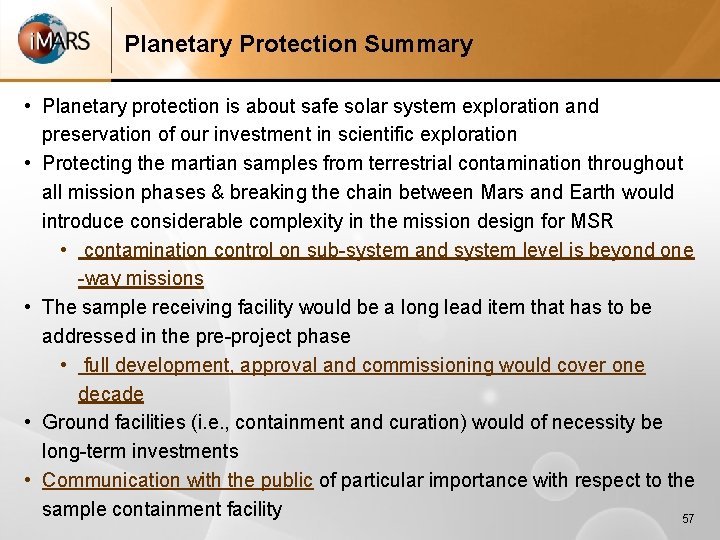
Planetary Protection Summary • Planetary protection is about safe solar system exploration and preservation of our investment in scientific exploration • Protecting the martian samples from terrestrial contamination throughout all mission phases & breaking the chain between Mars and Earth would introduce considerable complexity in the mission design for MSR • contamination control on sub-system and system level is beyond one -way missions • The sample receiving facility would be a long lead item that has to be addressed in the pre-project phase • full development, approval and commissioning would cover one decade • Ground facilities (i. e. , containment and curation) would of necessity be long-term investments • Communication with the public of particular importance with respect to the sample containment facility 57
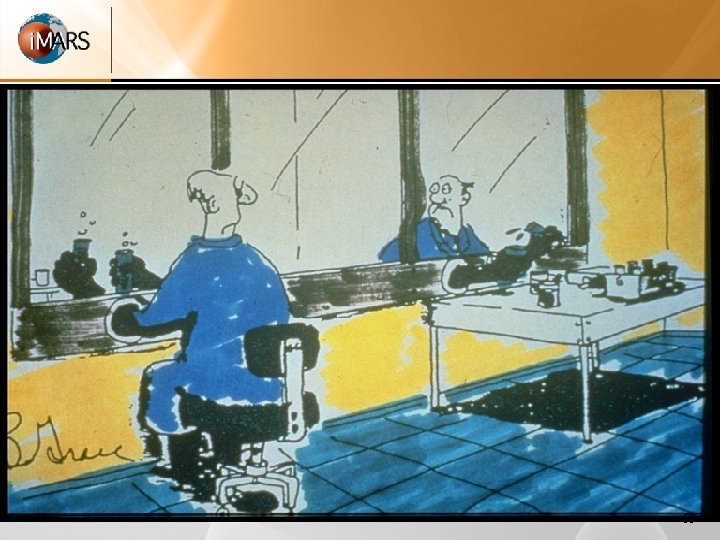
58
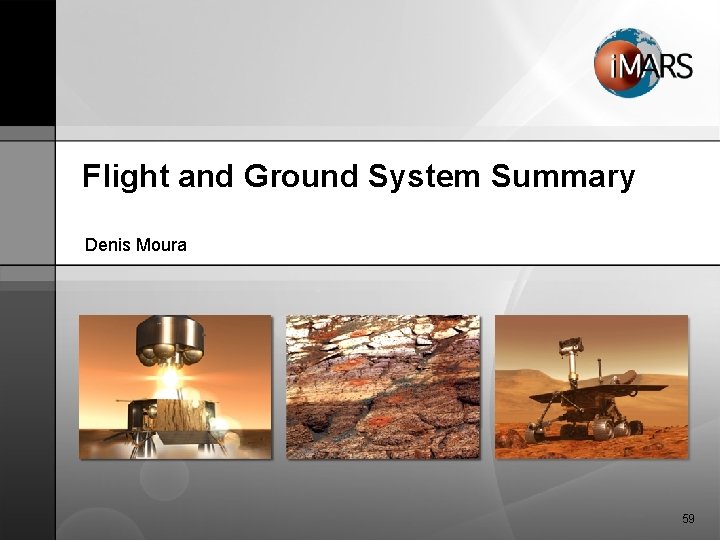
Flight and Ground System Summary Denis Moura 59
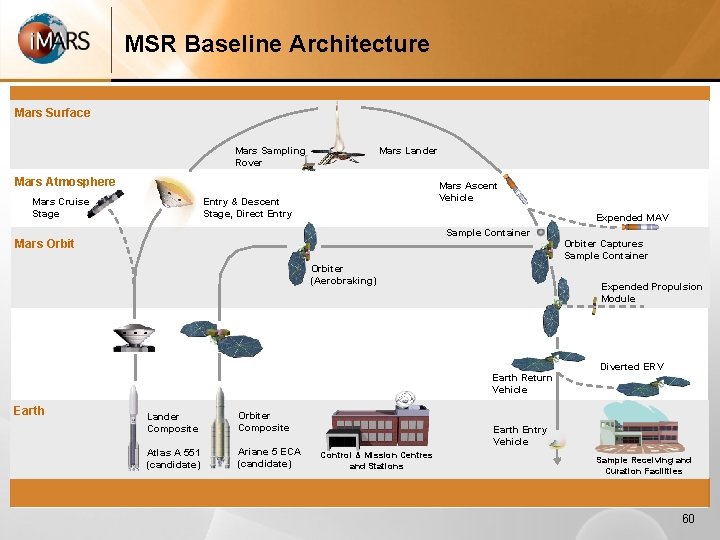
MSR Baseline Architecture Mars Surface Mars Sampling Rover Mars Lander Mars Atmosphere Mars Ascent Vehicle Entry & Descent Stage, Direct Entry Mars Cruise Stage Expended MAV Sample Container Mars Orbiter (Aerobraking) Expended Propulsion Module Earth Return Vehicle Earth Lander Composite Orbiter Composite Atlas A 551 (candidate) Ariane 5 ECA (candidate) Orbiter Captures Sample Container Diverted ERV Earth Entry Vehicle Control & Mission Centres and Stations Sample Receiving and Curation Facilities 60
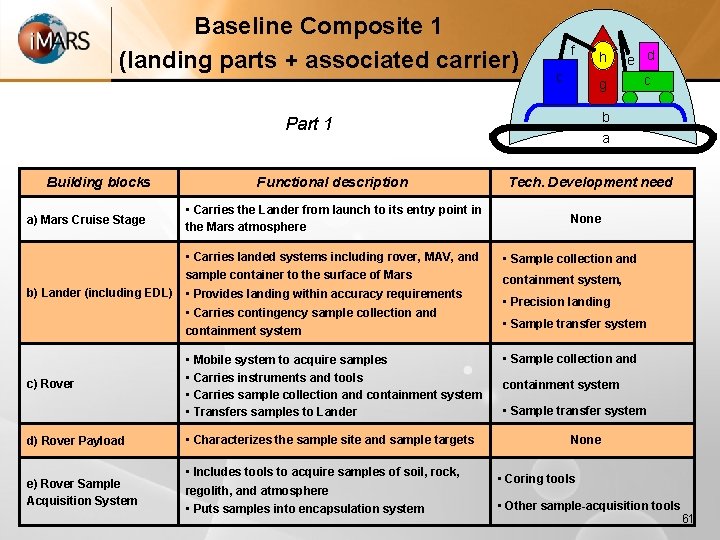
Baseline Composite 1 (landing parts + associated carrier) f c h g b Part 1 Building blocks a) Mars Cruise Stage Functional description • Carries the Lander from launch to its entry point in the Mars atmosphere e d c a Tech. Development need None • Carries landed systems including rover, MAV, and sample container to the surface of Mars b) Lander (including EDL) • Provides landing within accuracy requirements • Carries contingency sample collection and containment system • Sample collection and • Mobile system to acquire samples • Carries instruments and tools • Carries sample collection and containment system • Transfers samples to Lander • Sample collection and c) Rover d) Rover Payload • Characterizes the sample site and sample targets e) Rover Sample Acquisition System • Includes tools to acquire samples of soil, rock, regolith, and atmosphere • Puts samples into encapsulation system containment system, • Precision landing • Sample transfer system containment system • Sample transfer system None • Coring tools • Other sample-acquisition tools 61
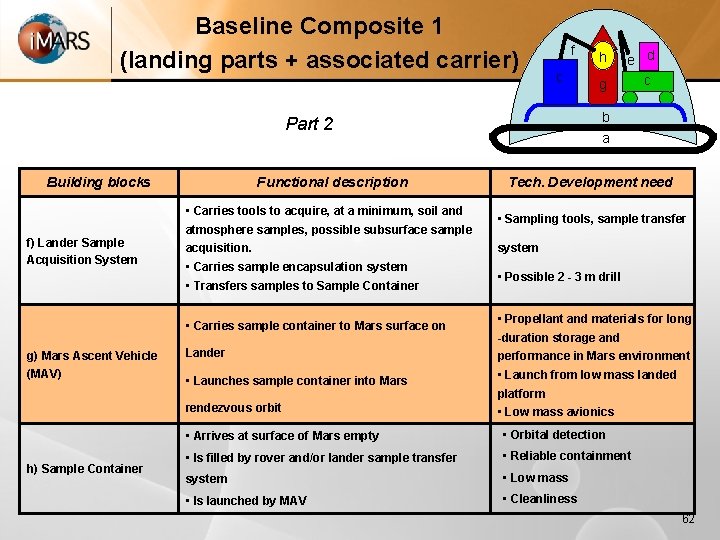
Baseline Composite 1 (landing parts + associated carrier) f c f) Lander Sample Acquisition System Functional description • Carries tools to acquire, at a minimum, soil and atmosphere samples, possible subsurface sample acquisition. • Carries sample encapsulation system • Transfers samples to Sample Container • Carries sample container to Mars surface on g) Mars Ascent Vehicle (MAV) Lander • Launches sample container into Mars rendezvous orbit h) Sample Container g e d c b Part 2 Building blocks h a Tech. Development need • Sampling tools, sample transfer system • Possible 2 - 3 m drill • Propellant and materials for long -duration storage and performance in Mars environment • Launch from low mass landed platform • Low mass avionics • Arrives at surface of Mars empty • Orbital detection • Is filled by rover and/or lander sample transfer • Reliable containment system • Low mass • Is launched by MAV • Cleanliness 62
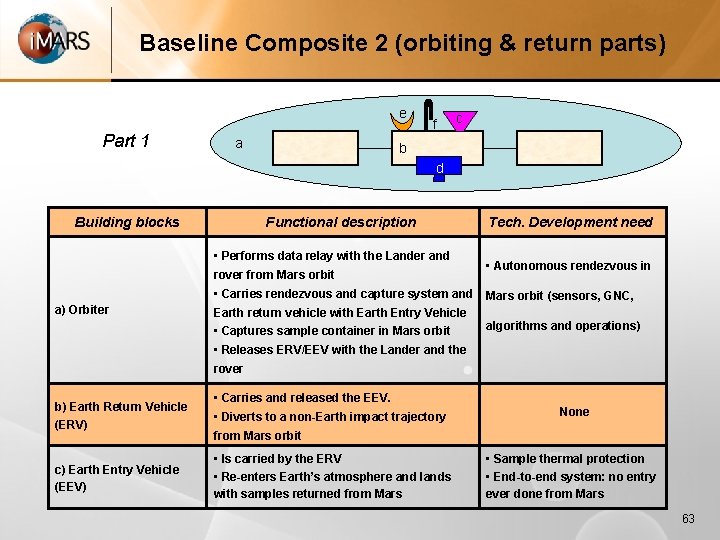
Baseline Composite 2 (orbiting & return parts) e Part 1 a f c b d Building blocks a) Orbiter Functional description • Performs data relay with the Lander and rover from Mars orbit • Carries rendezvous and capture system and Earth return vehicle with Earth Entry Vehicle • Captures sample container in Mars orbit • Releases ERV/EEV with the Lander and the rover Tech. Development need • Autonomous rendezvous in Mars orbit (sensors, GNC, algorithms and operations) b) Earth Return Vehicle (ERV) • Carries and released the EEV. • Diverts to a non-Earth impact trajectory from Mars orbit None c) Earth Entry Vehicle (EEV) • Is carried by the ERV • Re-enters Earth’s atmosphere and lands with samples returned from Mars • Sample thermal protection • End-to-end system: no entry ever done from Mars 63
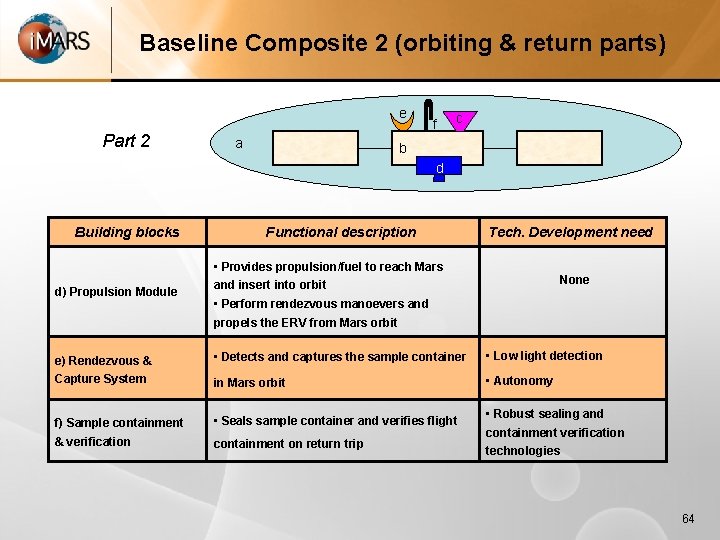
Baseline Composite 2 (orbiting & return parts) e Part 2 a f c b d Building blocks Functional description Tech. Development need d) Propulsion Module • Provides propulsion/fuel to reach Mars and insert into orbit • Perform rendezvous manoevers and propels the ERV from Mars orbit e) Rendezvous & • Detects and captures the sample container • Low light detection Capture System in Mars orbit • Autonomy f) Sample containment • Seals sample container and verifies flight & verification containment on return trip None • Robust sealing and containment verification technologies 64
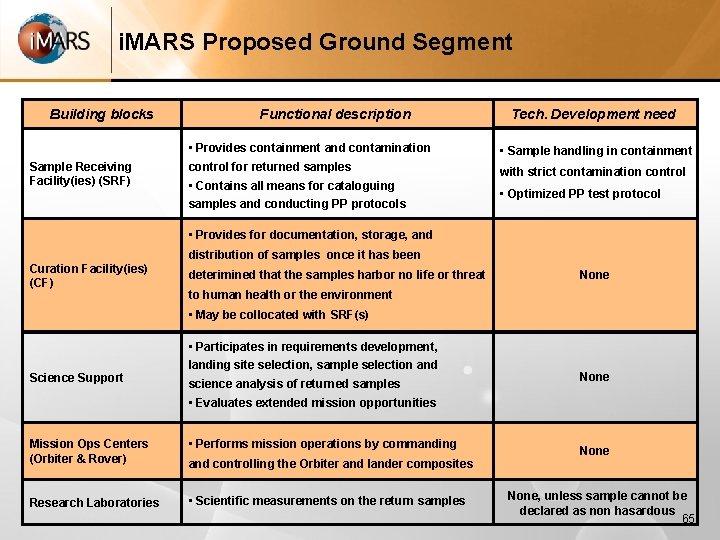
i. MARS Proposed Ground Segment Building blocks Sample Receiving Facility(ies) (SRF) Functional description • Provides containment and contamination control for returned samples • Contains all means for cataloguing samples and conducting PP protocols Tech. Development need • Sample handling in containment with strict contamination control • Optimized PP test protocol • Provides for documentation, storage, and distribution of samples once it has been Curation Facility(ies) (CF) deterimined that the samples harbor no life or threat None to human health or the environment • May be collocated with SRF(s) Science Support • Participates in requirements development, landing site selection, sample selection and science analysis of returned samples • Evaluates extended mission opportunities Mission Ops Centers (Orbiter & Rover) • Performs mission operations by commanding Research Laboratories • Scientific measurements on the return samples None and controlling the Orbiter and lander composites None, unless sample cannot be declared as non hasardous 65
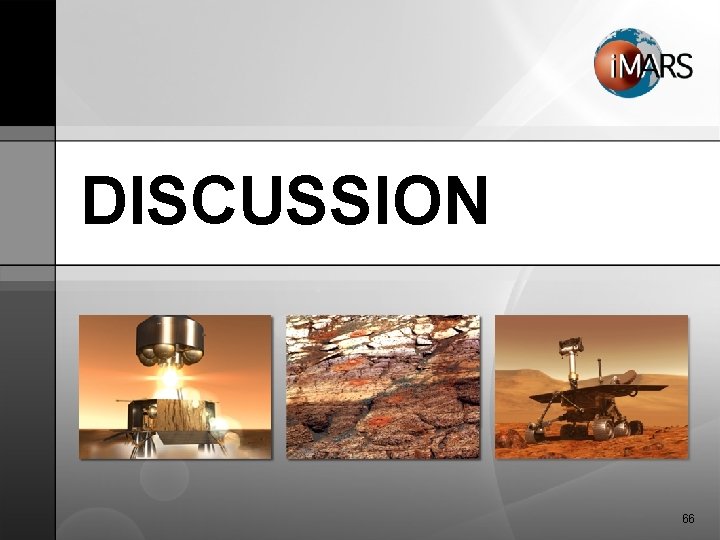
DISCUSSION 66
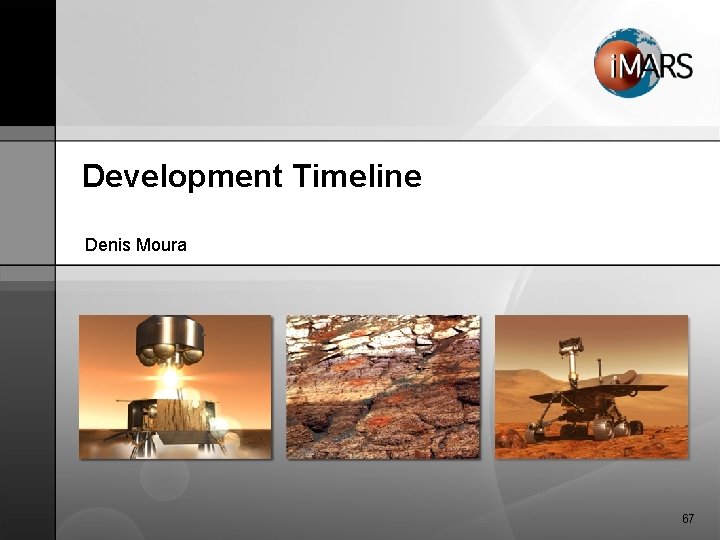
Development Timeline Denis Moura 67
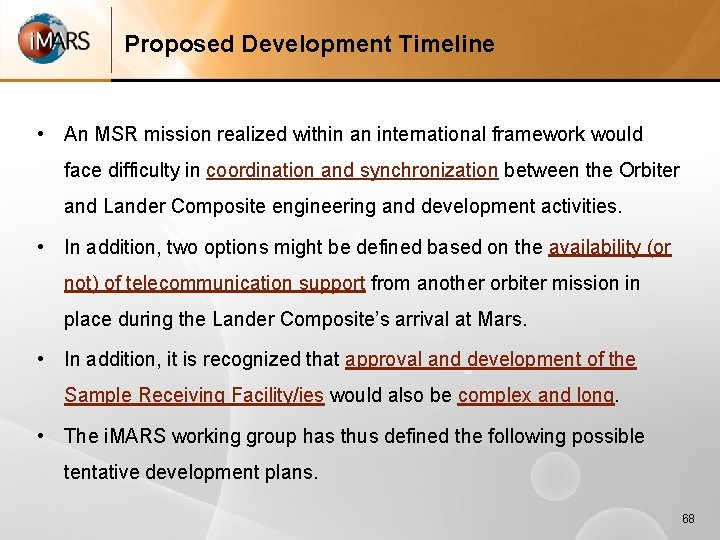
Proposed Development Timeline • An MSR mission realized within an international framework would face difficulty in coordination and synchronization between the Orbiter and Lander Composite engineering and development activities. • In addition, two options might be defined based on the availability (or not) of telecommunication support from another orbiter mission in place during the Lander Composite’s arrival at Mars. • In addition, it is recognized that approval and development of the Sample Receiving Facility/ies would also be complex and long. • The i. MARS working group has thus defined the following possible tentative development plans. 68
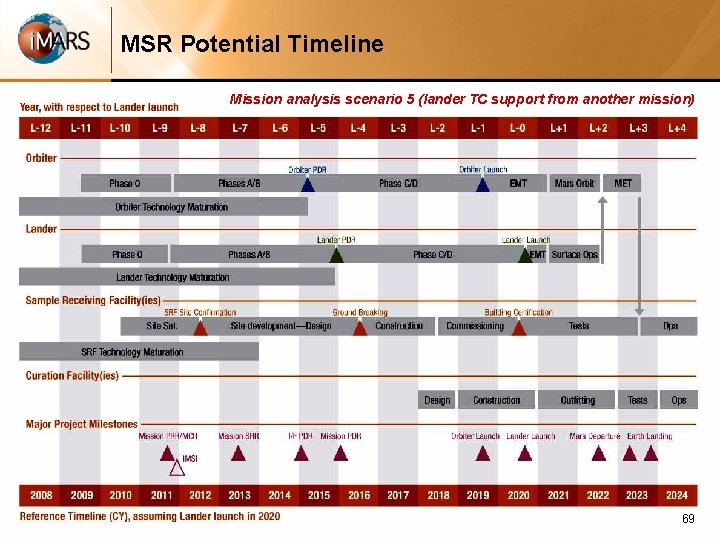
MSR Potential Timeline Mission analysis scenario 5 (lander TC support from another mission) 69
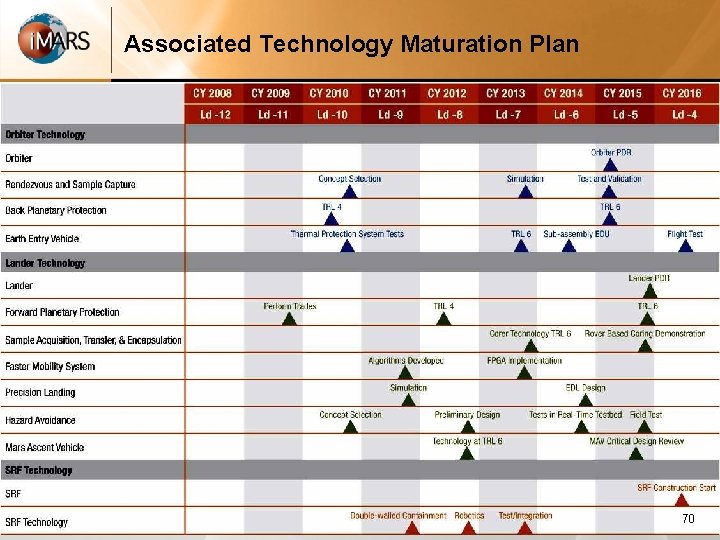
Associated Technology Maturation Plan 70
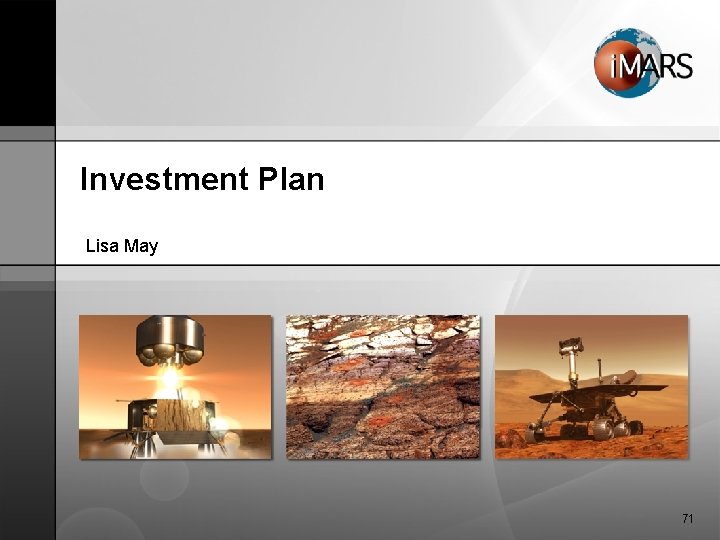
Investment Plan Lisa May 71
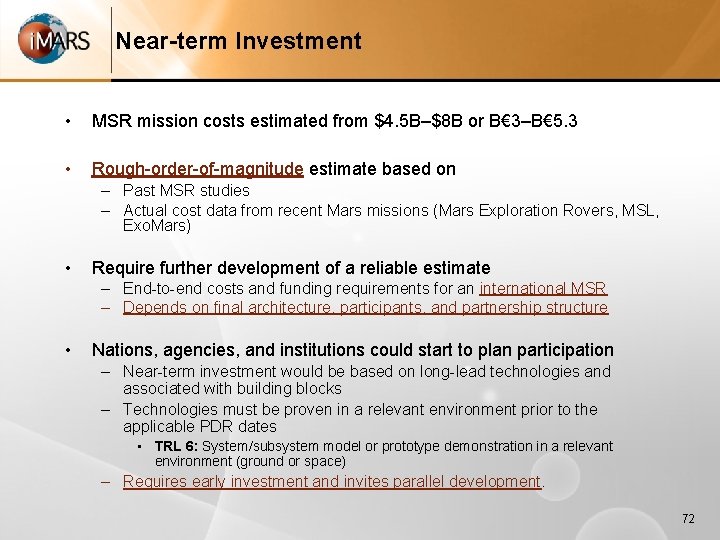
Near-term Investment • MSR mission costs estimated from $4. 5 B–$8 B or B€ 3–B€ 5. 3 • Rough-order-of-magnitude estimate based on – Past MSR studies – Actual cost data from recent Mars missions (Mars Exploration Rovers, MSL, Exo. Mars) • Require further development of a reliable estimate – End-to-end costs and funding requirements for an international MSR – Depends on final architecture, participants, and partnership structure • Nations, agencies, and institutions could start to plan participation – Near-term investment would be based on long-lead technologies and associated with building blocks – Technologies must be proven in a relevant environment prior to the applicable PDR dates • TRL 6: System/subsystem model or prototype demonstration in a relevant environment (ground or space) – Requires early investment and invites parallel development. 72
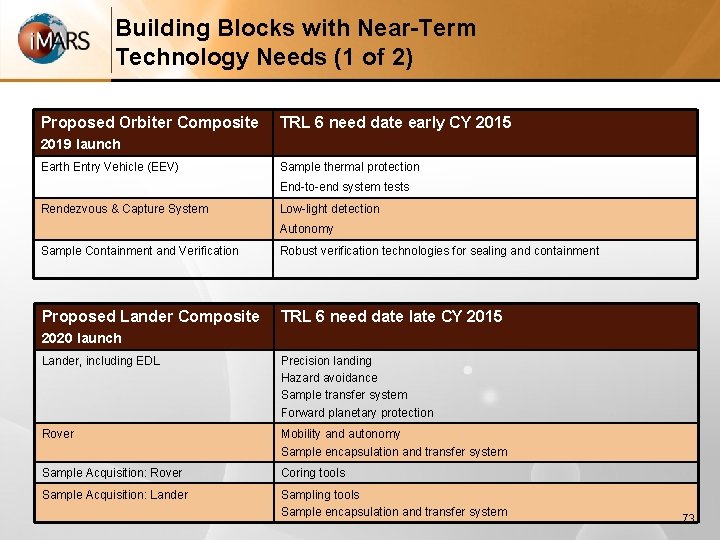
Building Blocks with Near-Term Technology Needs (1 of 2) Proposed Orbiter Composite TRL 6 need date early CY 2015 2019 launch Earth Entry Vehicle (EEV) Sample thermal protection End-to-end system tests Rendezvous & Capture System Low-light detection Autonomy Sample Containment and Verification Robust verification technologies for sealing and containment Proposed Lander Composite TRL 6 need date late CY 2015 2020 launch Lander, including EDL Precision landing Hazard avoidance Sample transfer system Forward planetary protection Rover Mobility and autonomy Sample encapsulation and transfer system Sample Acquisition: Rover Coring tools Sample Acquisition: Lander Sampling tools Sample encapsulation and transfer system 73
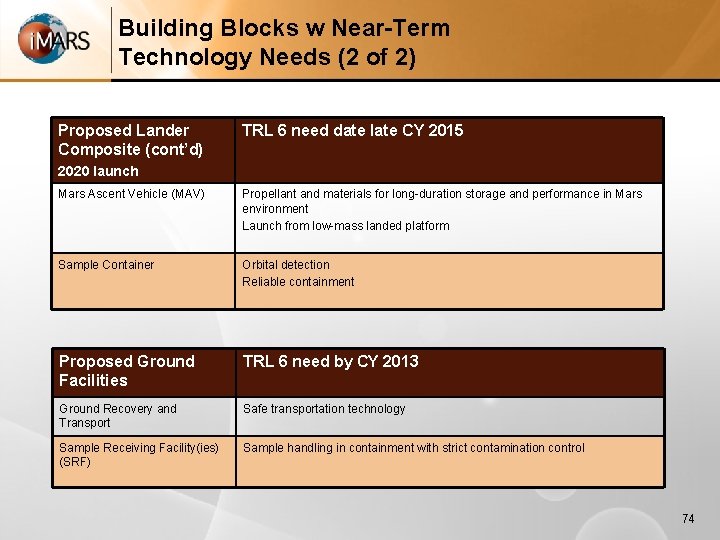
Building Blocks w Near-Term Technology Needs (2 of 2) Proposed Lander Composite (cont’d) TRL 6 need date late CY 2015 2020 launch Mars Ascent Vehicle (MAV) Propellant and materials for long-duration storage and performance in Mars environment Launch from low-mass landed platform Sample Container Orbital detection Reliable containment Proposed Ground Facilities TRL 6 need by CY 2013 Ground Recovery and Transport Safe transportation technology Sample Receiving Facility(ies) (SRF) Sample handling in containment with strict contamination control 74
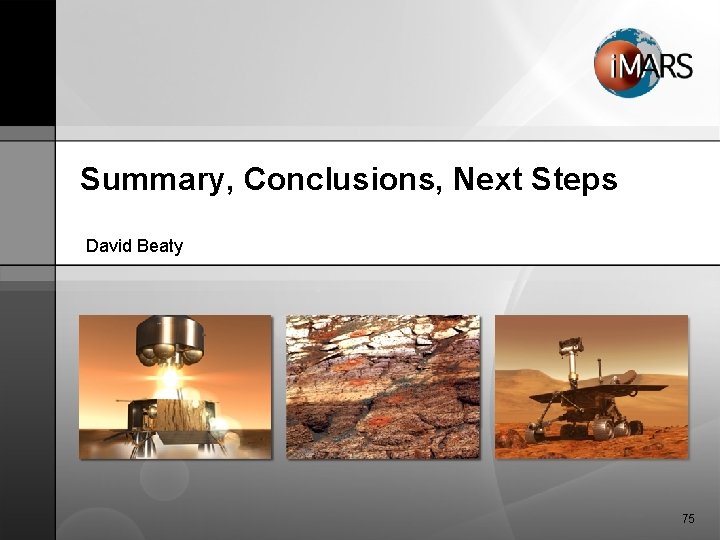
Summary, Conclusions, Next Steps David Beaty 75
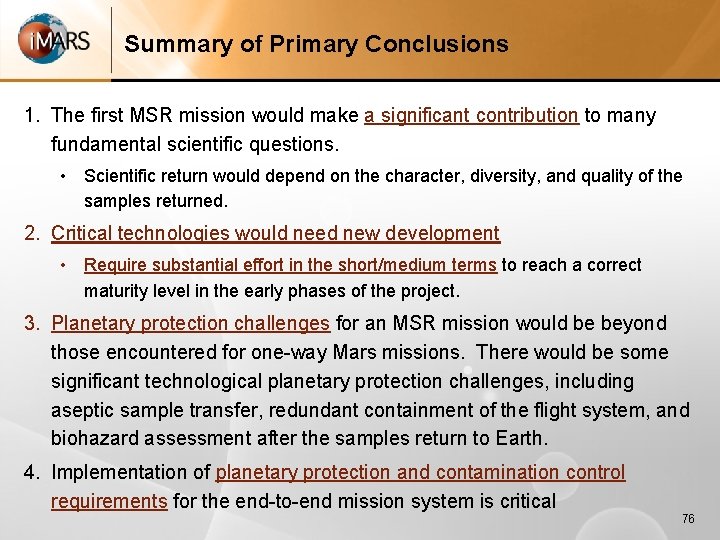
Summary of Primary Conclusions 1. The first MSR mission would make a significant contribution to many fundamental scientific questions. • Scientific return would depend on the character, diversity, and quality of the samples returned. 2. Critical technologies would need new development • Require substantial effort in the short/medium terms to reach a correct maturity level in the early phases of the project. 3. Planetary protection challenges for an MSR mission would be beyond those encountered for one-way Mars missions. There would be some significant technological planetary protection challenges, including aseptic sample transfer, redundant containment of the flight system, and biohazard assessment after the samples return to Earth. 4. Implementation of planetary protection and contamination control requirements for the end-to-end mission system is critical 76
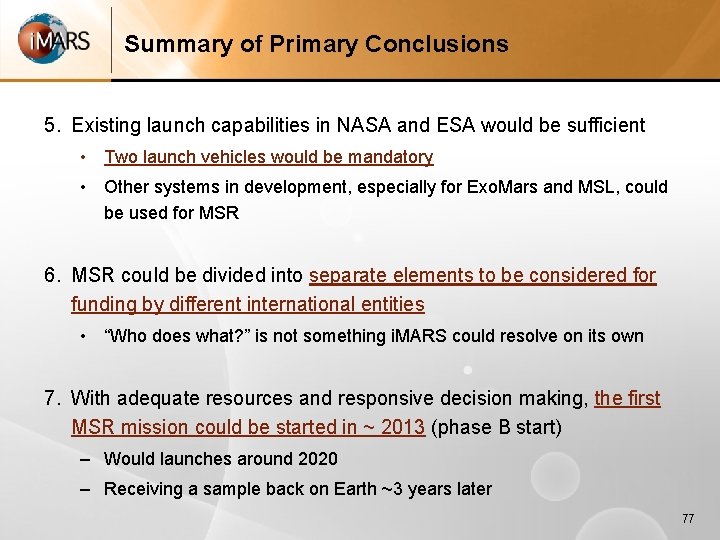
Summary of Primary Conclusions 5. Existing launch capabilities in NASA and ESA would be sufficient • Two launch vehicles would be mandatory • Other systems in development, especially for Exo. Mars and MSL, could be used for MSR 6. MSR could be divided into separate elements to be considered for funding by different international entities • “Who does what? ” is not something i. MARS could resolve on its own 7. With adequate resources and responsive decision making, the first MSR mission could be started in ~ 2013 (phase B start) – Would launches around 2020 – Receiving a sample back on Earth ~3 years later 77
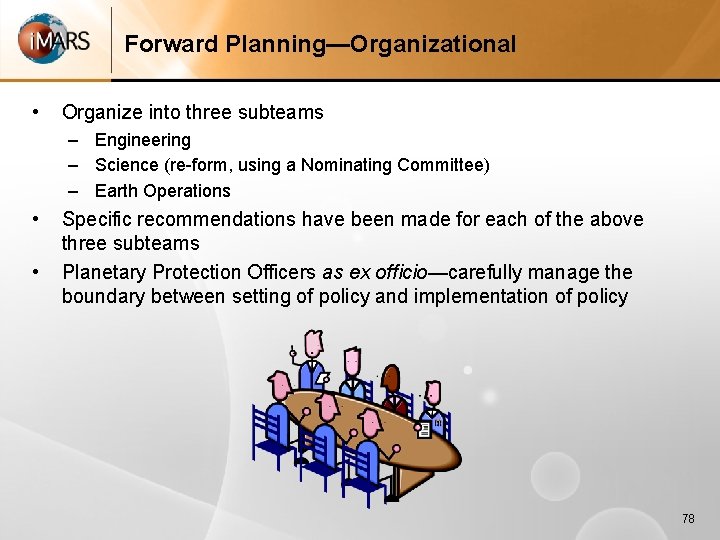
Forward Planning—Organizational • Organize into three subteams – Engineering – Science (re-form, using a Nominating Committee) – Earth Operations • • Specific recommendations have been made for each of the above three subteams Planetary Protection Officers as ex officio—carefully manage the boundary between setting of policy and implementation of policy 78
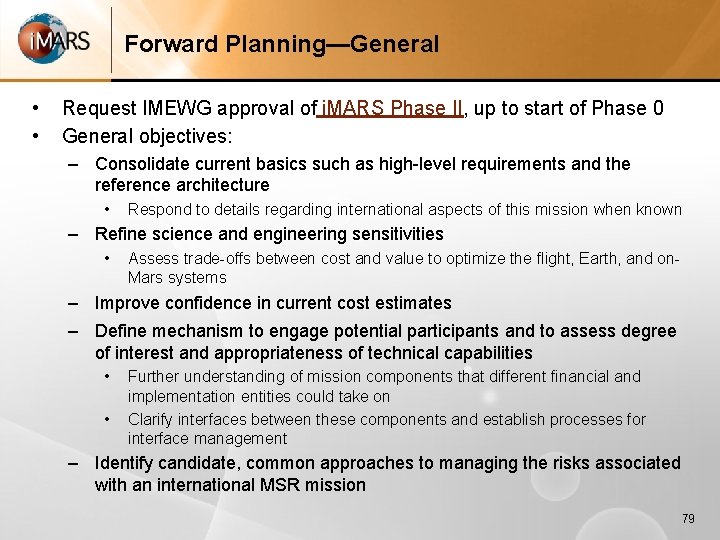
Forward Planning—General • • Request IMEWG approval of i. MARS Phase II, up to start of Phase 0 General objectives: – Consolidate current basics such as high-level requirements and the reference architecture • Respond to details regarding international aspects of this mission when known – Refine science and engineering sensitivities • Assess trade-offs between cost and value to optimize the flight, Earth, and on. Mars systems – Improve confidence in current cost estimates – Define mechanism to engage potential participants and to assess degree of interest and appropriateness of technical capabilities • • Further understanding of mission components that different financial and implementation entities could take on Clarify interfaces between these components and establish processes for interface management – Identify candidate, common approaches to managing the risks associated with an international MSR mission 79
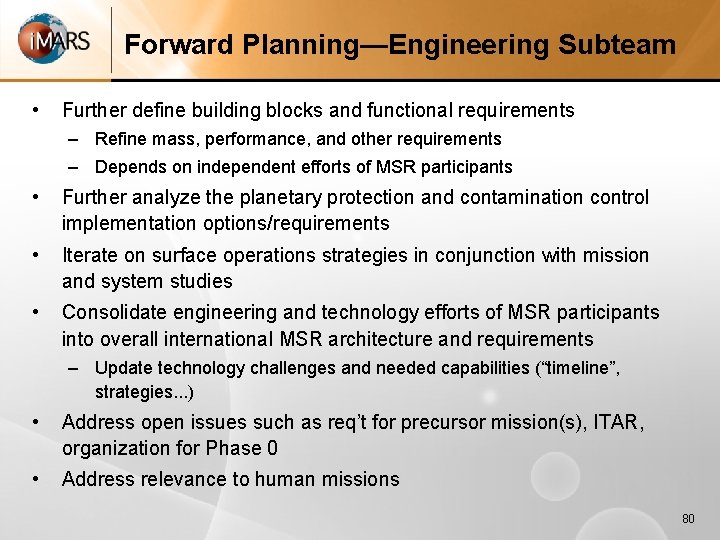
Forward Planning—Engineering Subteam • Further define building blocks and functional requirements – Refine mass, performance, and other requirements – Depends on independent efforts of MSR participants • Further analyze the planetary protection and contamination control implementation options/requirements • Iterate on surface operations strategies in conjunction with mission and system studies • Consolidate engineering and technology efforts of MSR participants into overall international MSR architecture and requirements – Update technology challenges and needed capabilities (“timeline”, strategies. . . ) • Address open issues such as req’t for precursor mission(s), ITAR, organization for Phase 0 • Address relevance to human missions 80
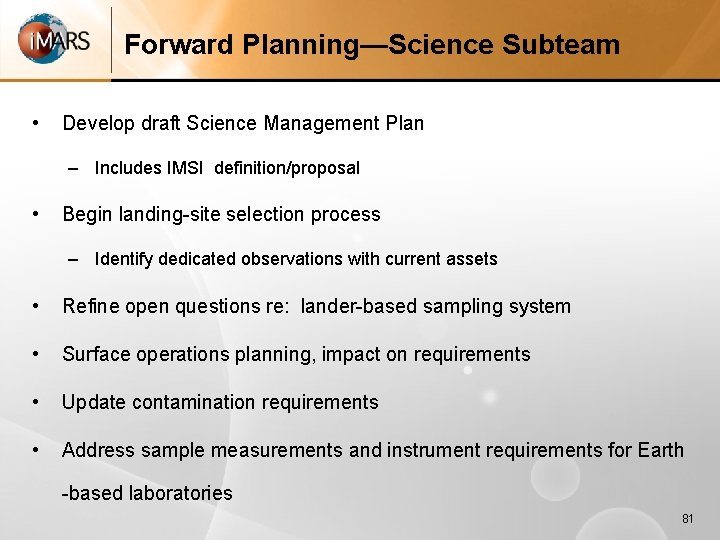
Forward Planning—Science Subteam • Develop draft Science Management Plan – Includes IMSI definition/proposal • Begin landing-site selection process – Identify dedicated observations with current assets • Refine open questions re: lander-based sampling system • Surface operations planning, impact on requirements • Update contamination requirements • Address sample measurements and instrument requirements for Earth -based laboratories 81
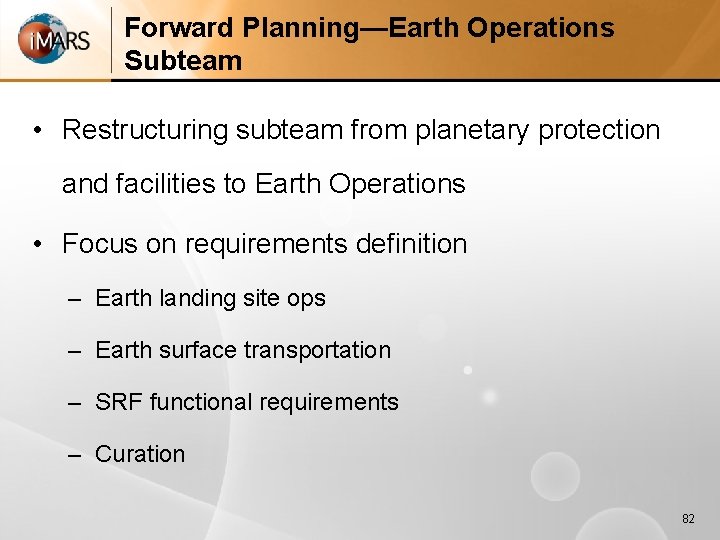
Forward Planning—Earth Operations Subteam • Restructuring subteam from planetary protection and facilities to Earth Operations • Focus on requirements definition – Earth landing site ops – Earth surface transportation – SRF functional requirements – Curation 82
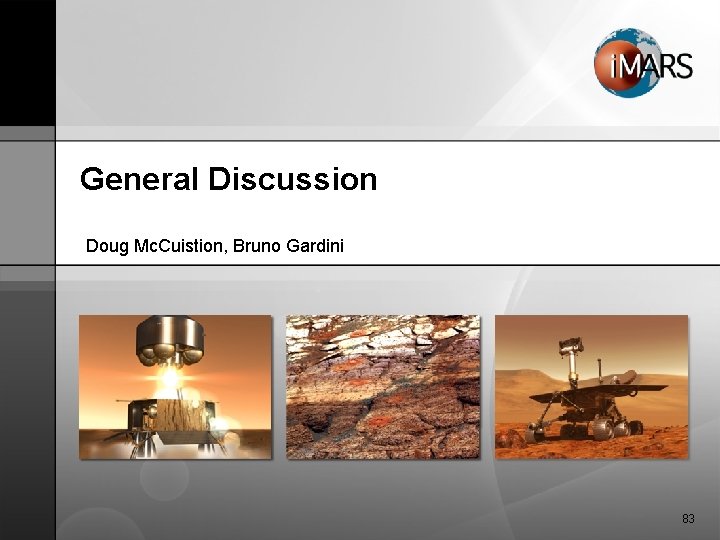
General Discussion Doug Mc. Cuistion, Bruno Gardini 83
Daily ward statement
Preliminary budget
Preliminary treatment adalah
Preliminary survey audit
P 波
Preliminary design review example
Preliminary materials
Mla rough draft example
Preliminary interview
Preliminary information gathering
Wilson company prepared the following preliminary budget
Ano ang pinakaunang pahina ng pamanahong papel
Preliminary graphics examples
Systems development life cycle
Objective thesis statement
Primary marksmanship instruction
Arrt preliminary score change
Image strategies
“gauge” refers to what quality of the film strip?
Determine junktrader’s preliminary net income.
Single flat wrap perm
Preliminary business plan
Preliminary prototype
Preliminary audit strategy
Preliminary outline
Pre softening milady
Fine grained screening
Ihrm training and development
Preliminary objectives
Advertising research process
Preliminary statement meaning
ñporo
Preliminary materiality
Haccp stands for
Preliminary marketing plan
Pdr preliminary design review
First aid pdhpe
Thesis statement fill in the blank
Preliminary ruling
Preliminary design review template
Recruitment and selection introduction
Preliminary works cited
Preliminary research
Reading pet part 2
Preliminary results example
Preliminary cooking and flavoring
Preliminary investigation
Preliminary assessment
Preliminary test
Preliminary outline speech
Fspos
Typiska novell drag
Nationell inriktning för artificiell intelligens
Returpilarna
Varför kallas perioden 1918-1939 för mellankrigstiden
En lathund för arbete med kontinuitetshantering
Adressändring ideell förening
Personlig tidbok fylla i
Sura för anatom
Densitet vatten
Datorkunskap för nybörjare
Stig kerman
Hur skriver man en tes
Autokratiskt ledarskap
Nyckelkompetenser för livslångt lärande
Påbyggnader för flakfordon
Lufttryck formel
Svenskt ramverk för digital samverkan
Kyssande vind analys
Presentera för publik crossboss
Jiddisch
Vem räknas som jude
Treserva lathund
Epiteltyper
Bästa kameran för astrofoto
Cks
Programskede byggprocessen
Bra mat för unga idrottare
Verktyg för automatisering av utbetalningar
Rutin för avvikelsehantering
Smärtskolan kunskap för livet
Ministerstyre för och nackdelar
Tack för att ni har lyssnat
Mall för referat