Nuclear Magnetic Resonance NMR Spectroscopy 1 NMR NUCLEAR
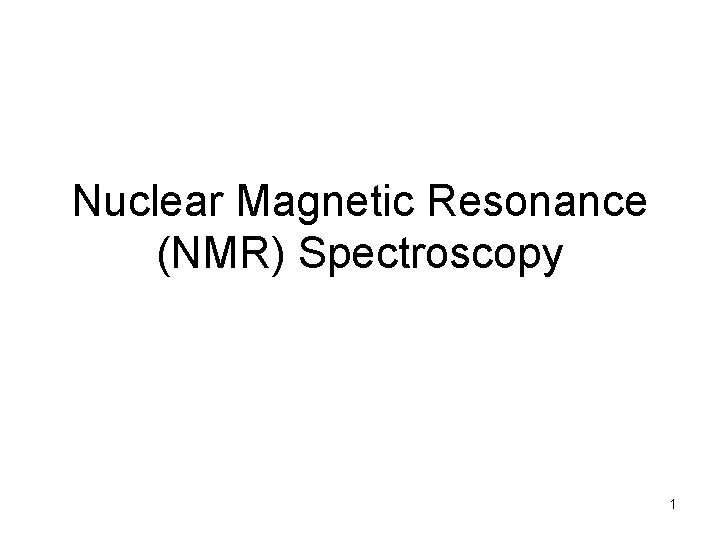
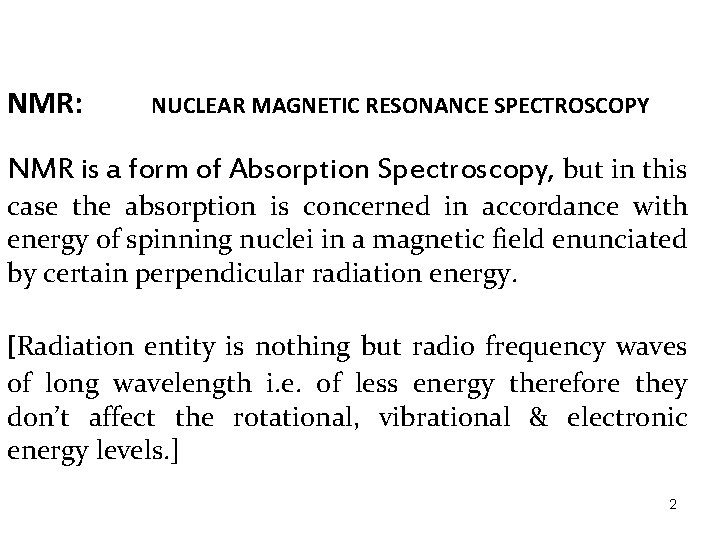
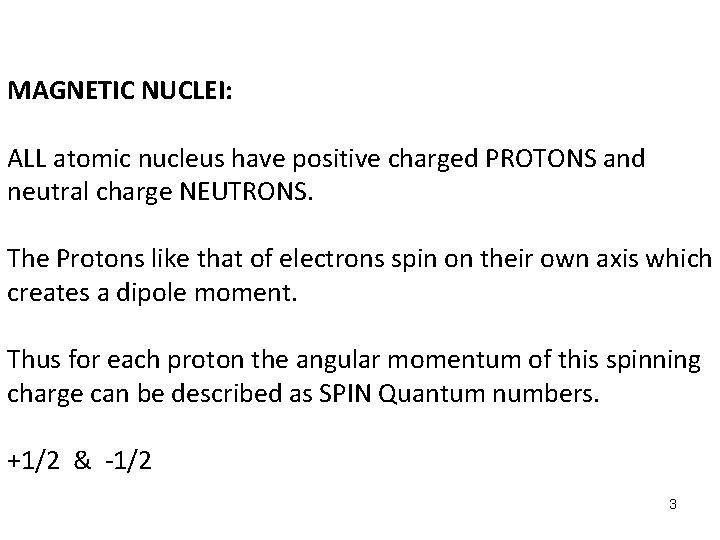
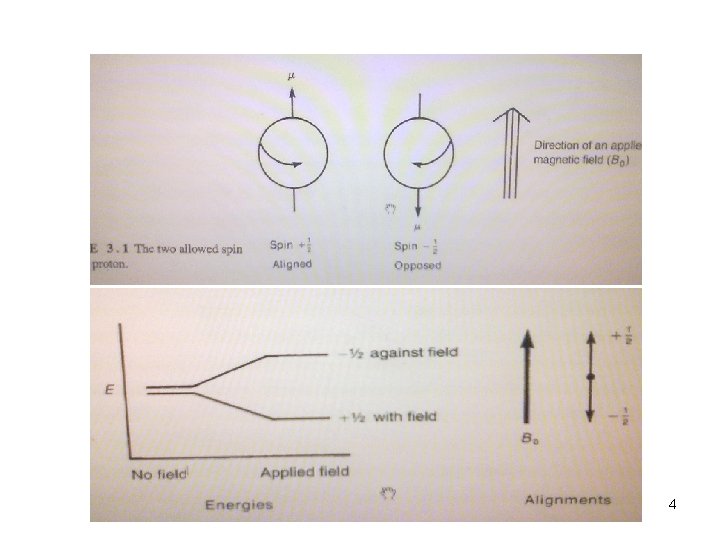
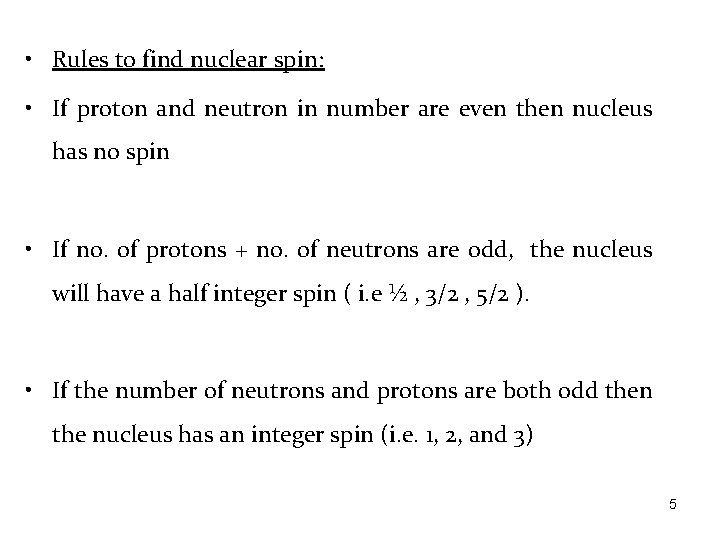
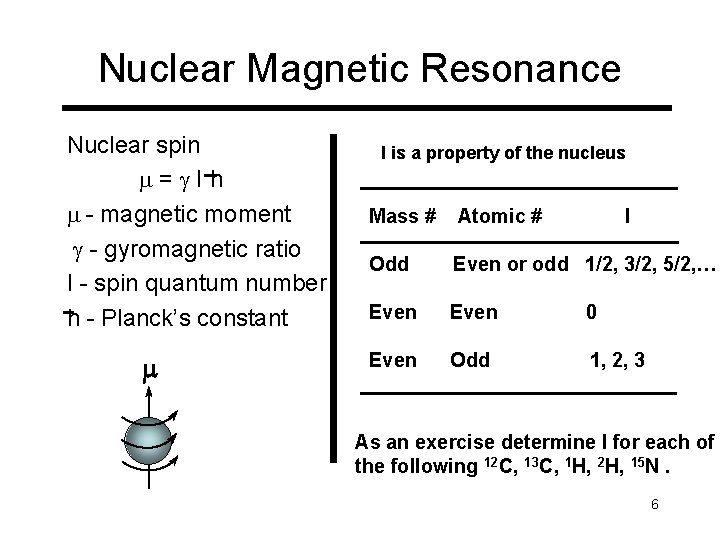
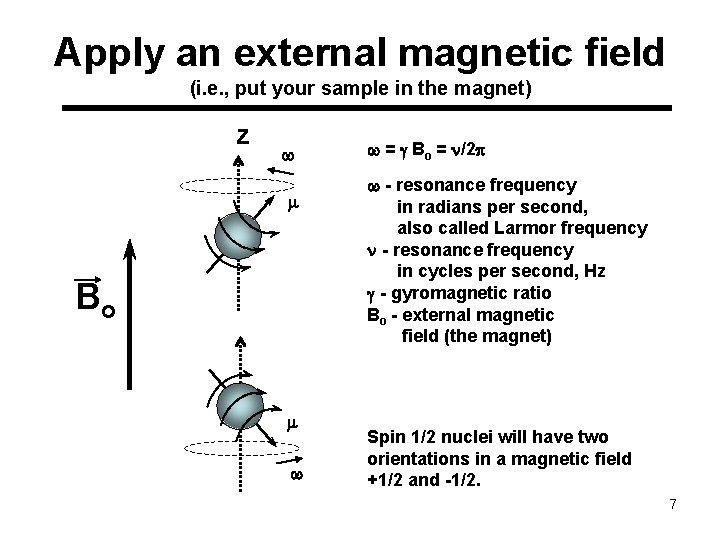
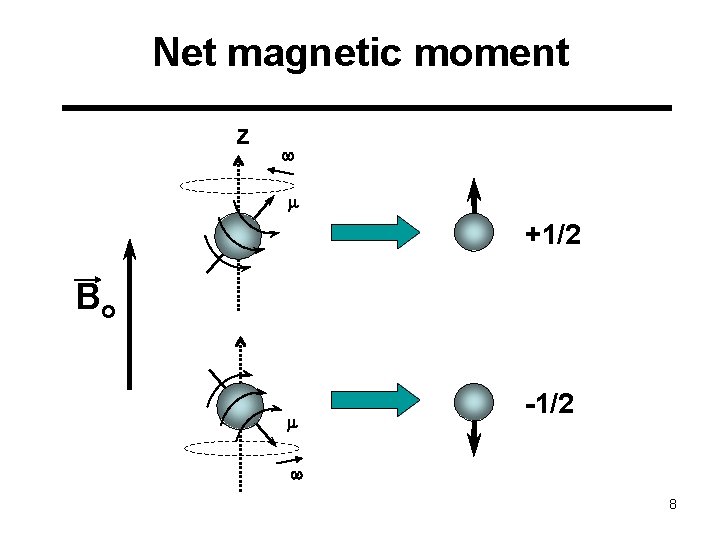
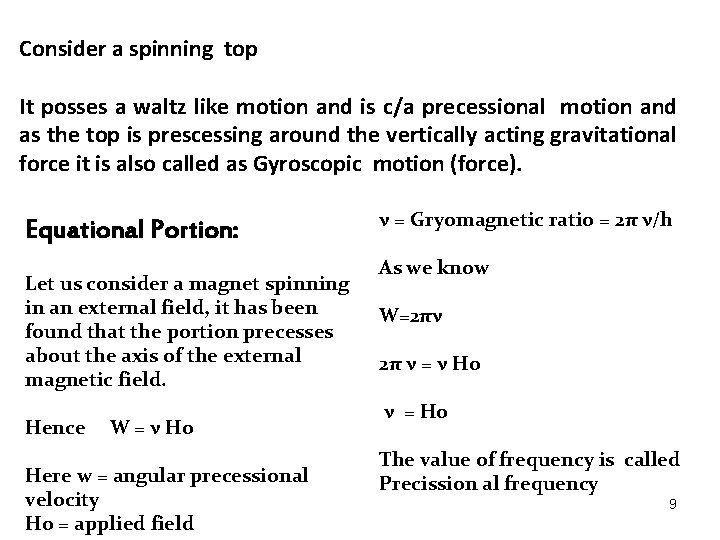
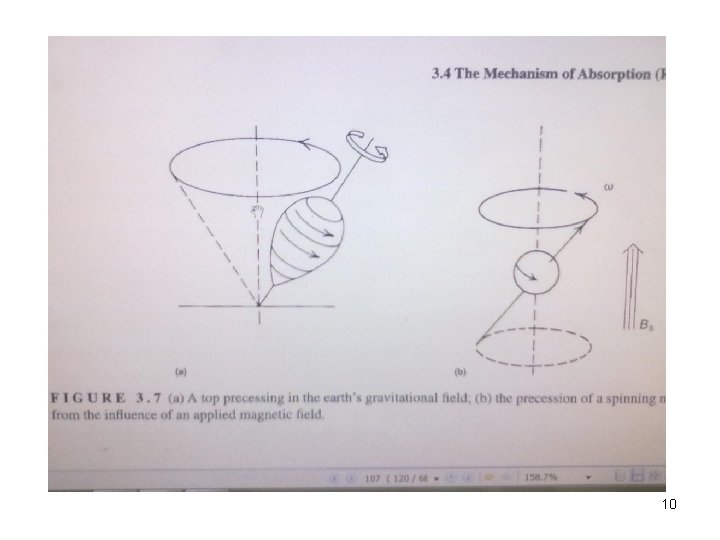
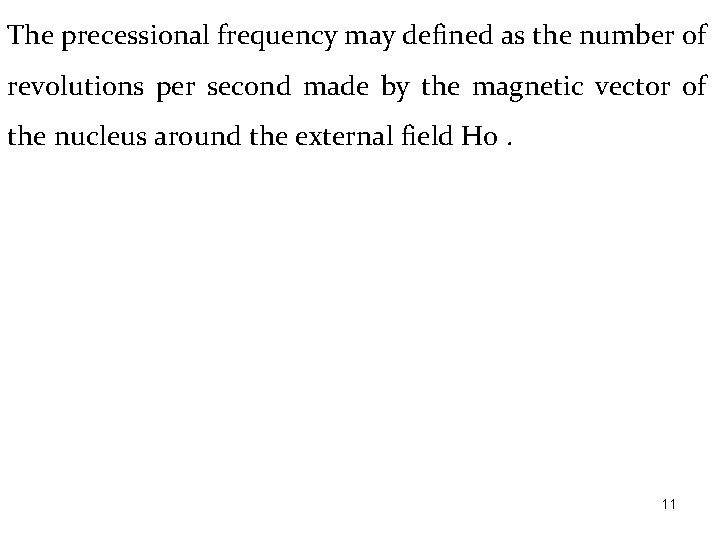
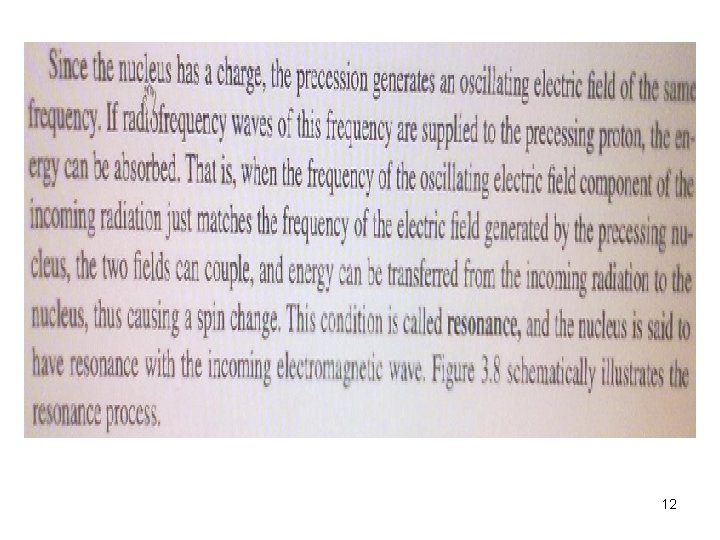
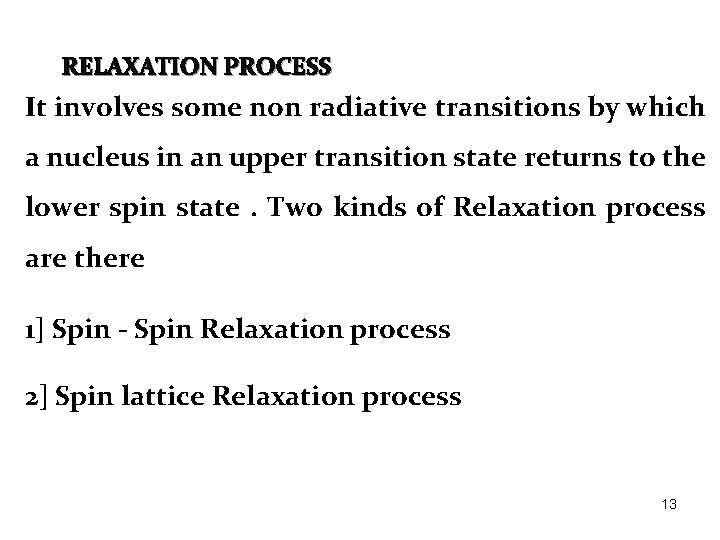
![1] Spin - Spin Relaxation process (transverse) It is due to mutant exchange of 1] Spin - Spin Relaxation process (transverse) It is due to mutant exchange of](https://slidetodoc.com/presentation_image_h2/76ce4179f00634e0da04039fc9d9f555/image-14.jpg)
![2 ] Spin lattice Relaxation process (Longitudinal) It involves the transfer of energy from 2 ] Spin lattice Relaxation process (Longitudinal) It involves the transfer of energy from](https://slidetodoc.com/presentation_image_h2/76ce4179f00634e0da04039fc9d9f555/image-15.jpg)
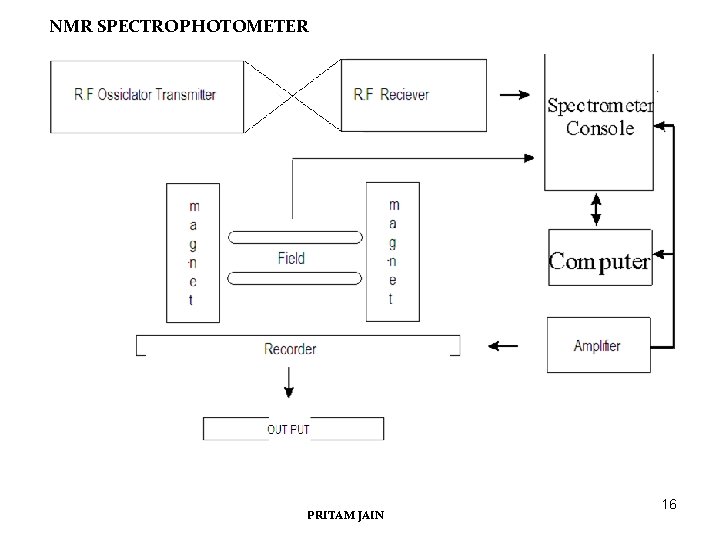
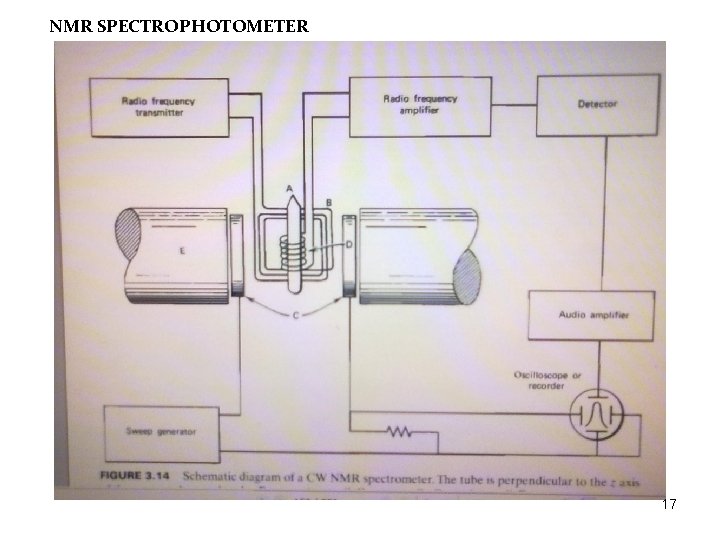
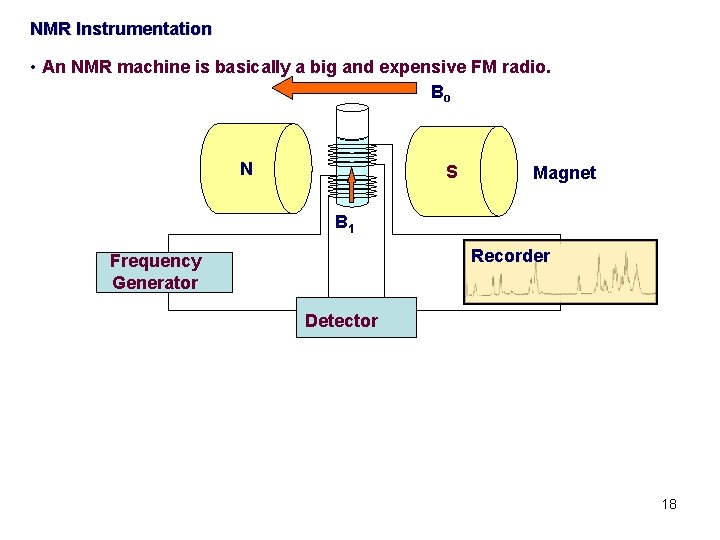
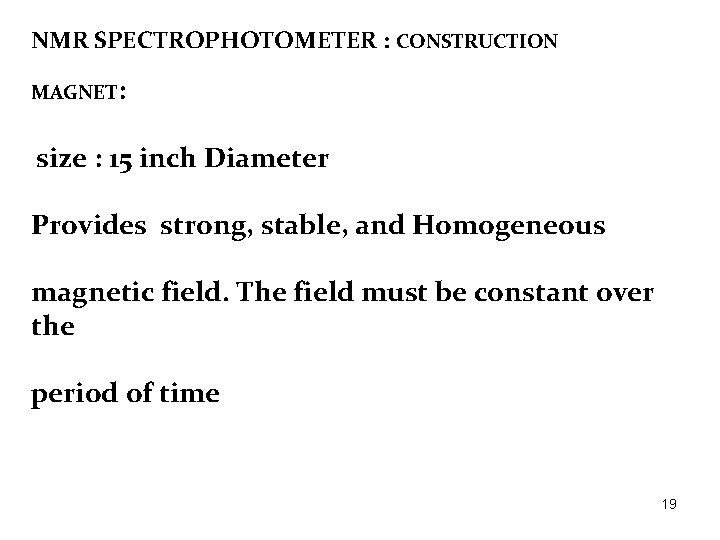
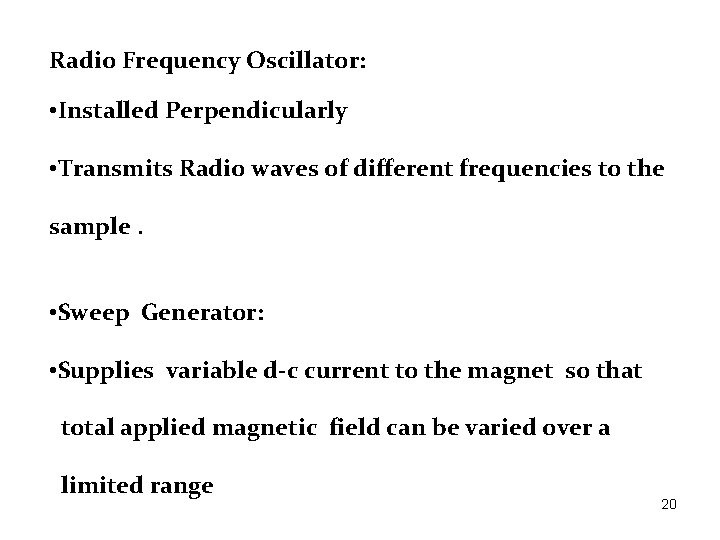
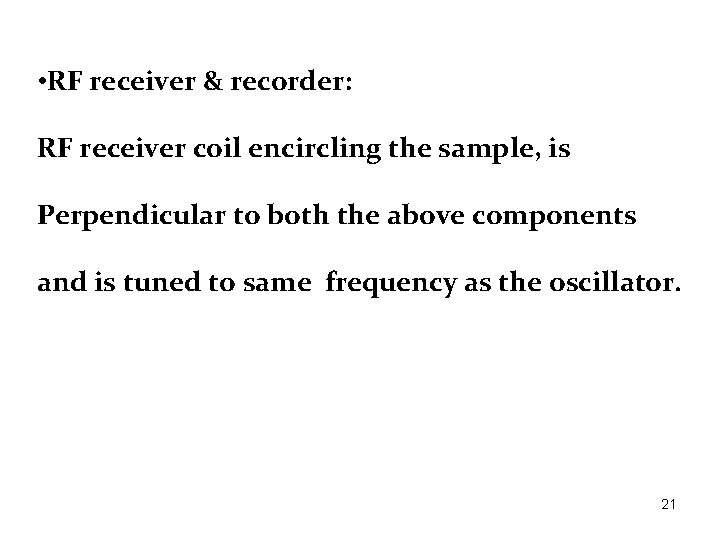
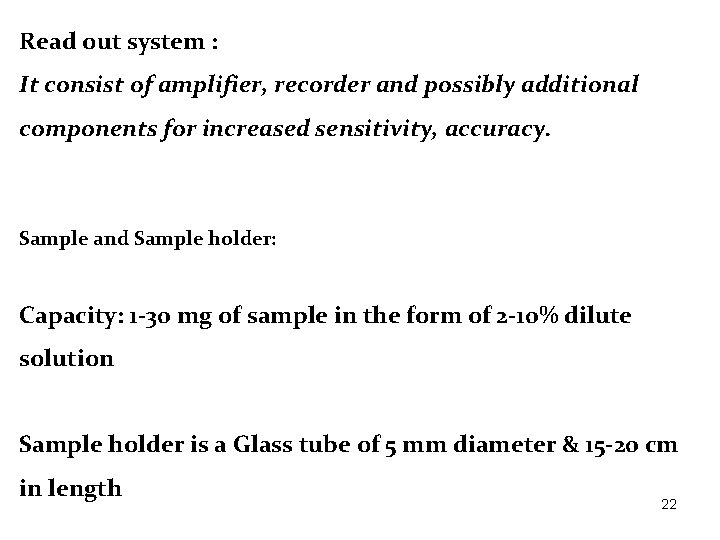
![TMS [tetra methyl silane (TMS (CH 3)4 Si)] is used in internal standard because: TMS [tetra methyl silane (TMS (CH 3)4 Si)] is used in internal standard because:](https://slidetodoc.com/presentation_image_h2/76ce4179f00634e0da04039fc9d9f555/image-23.jpg)
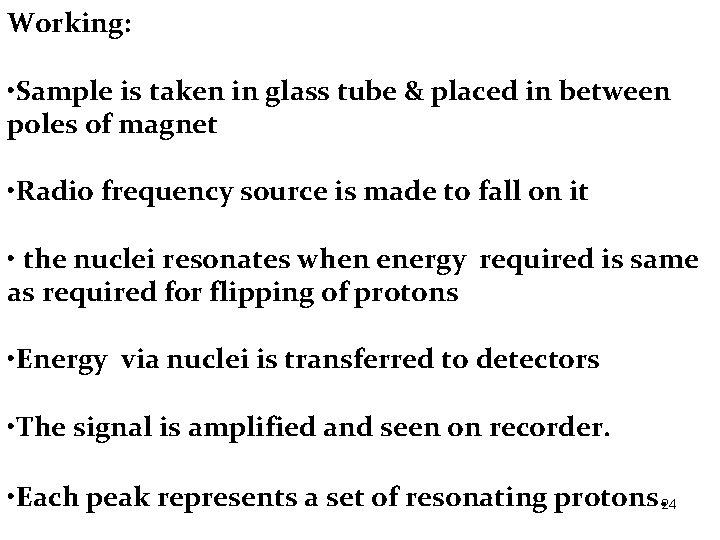
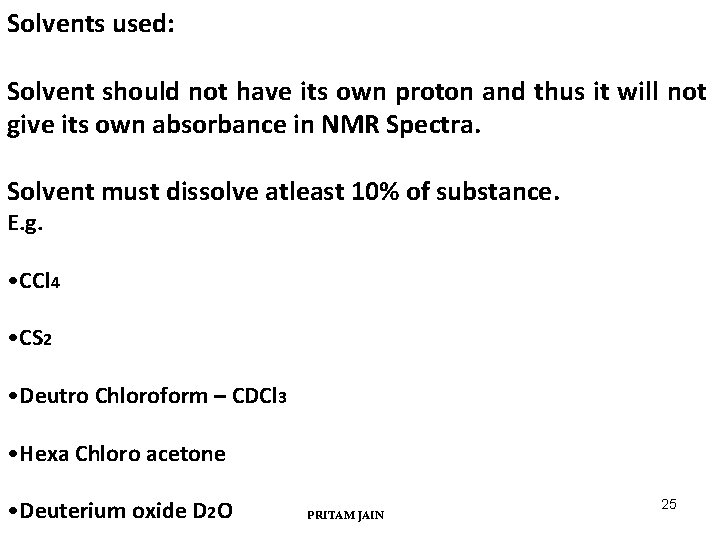
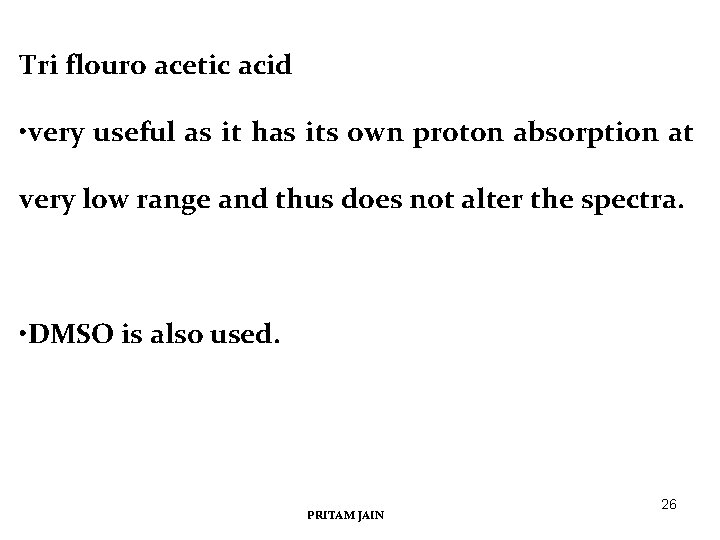
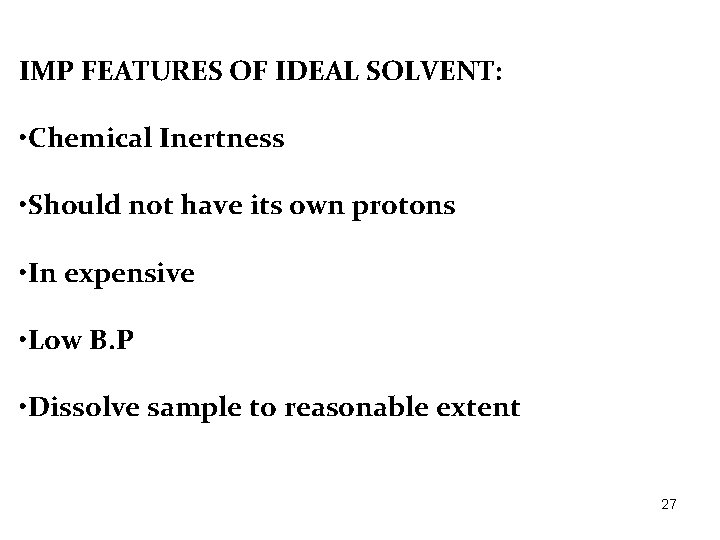
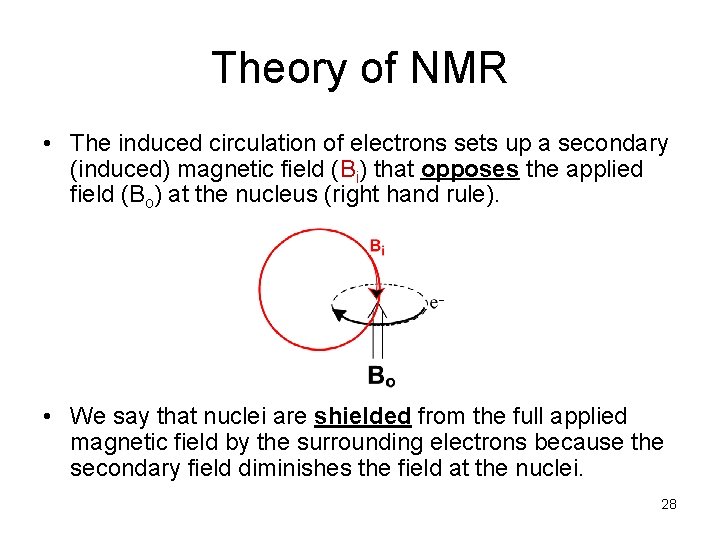
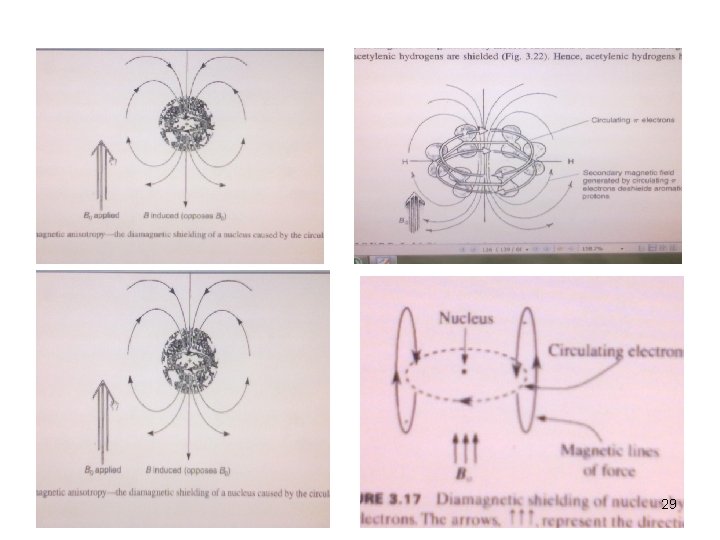
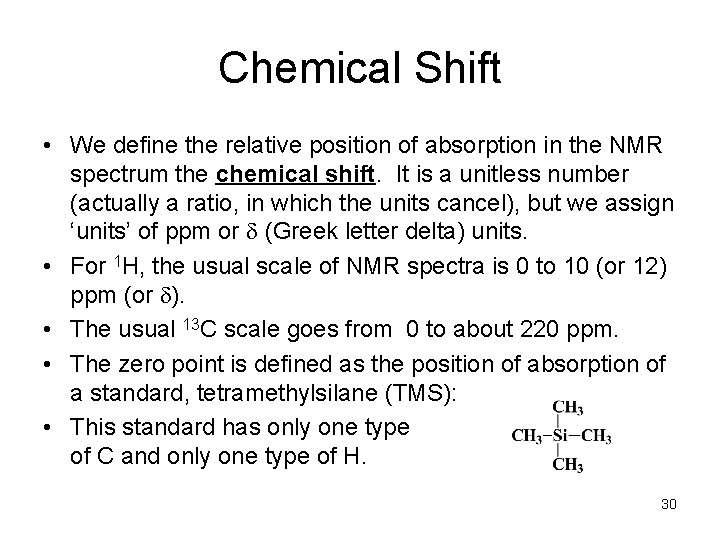
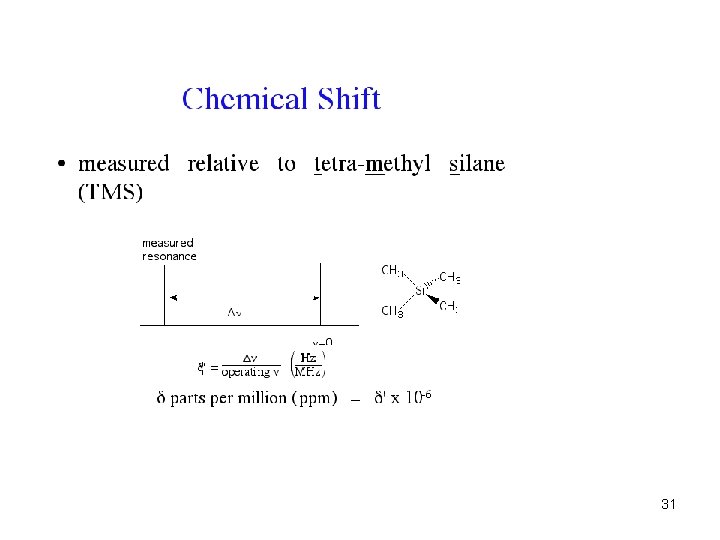
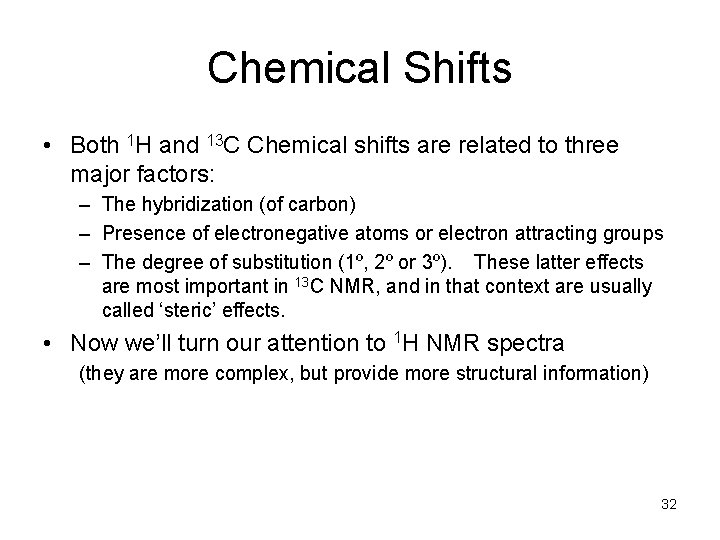
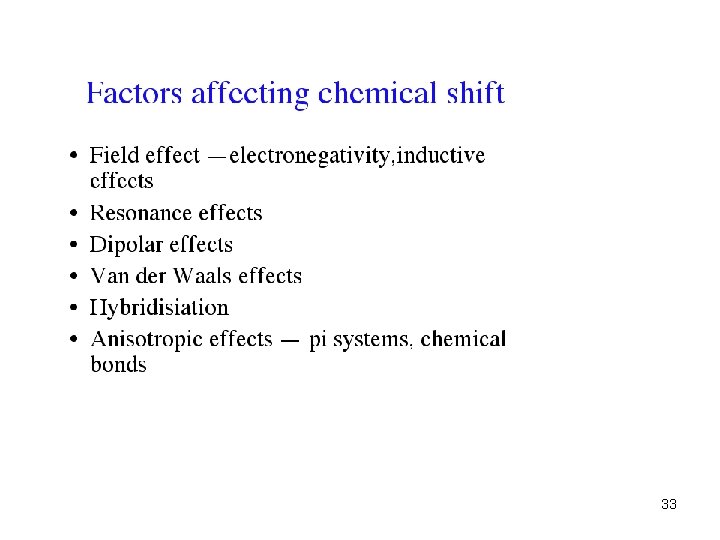
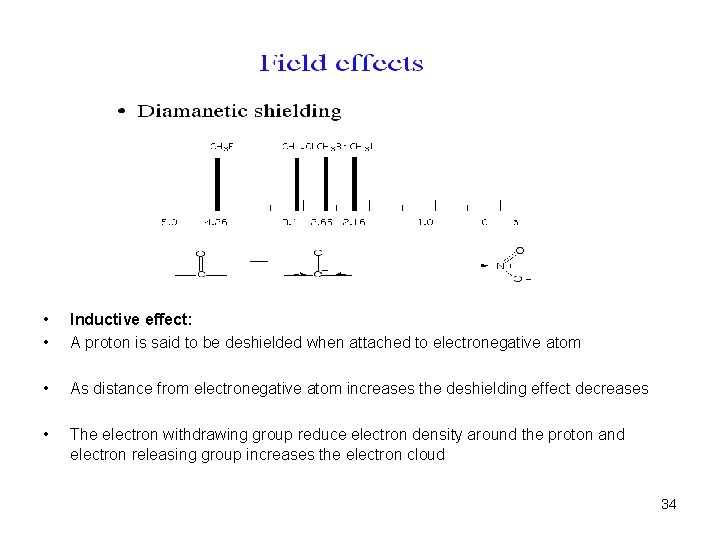
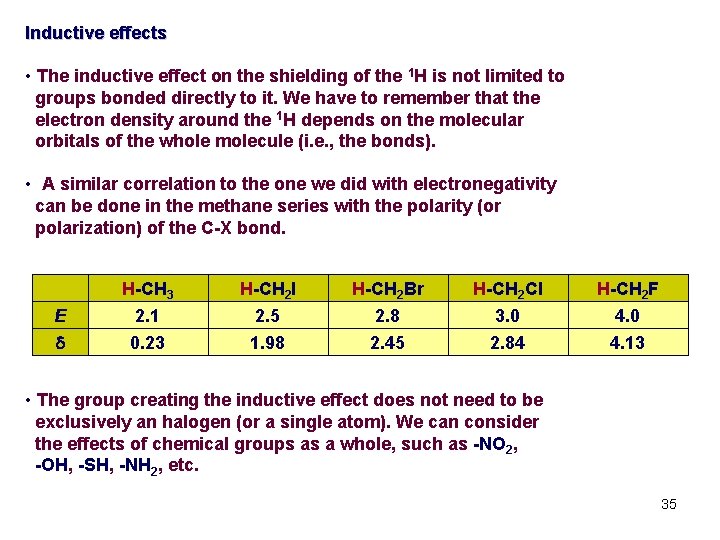
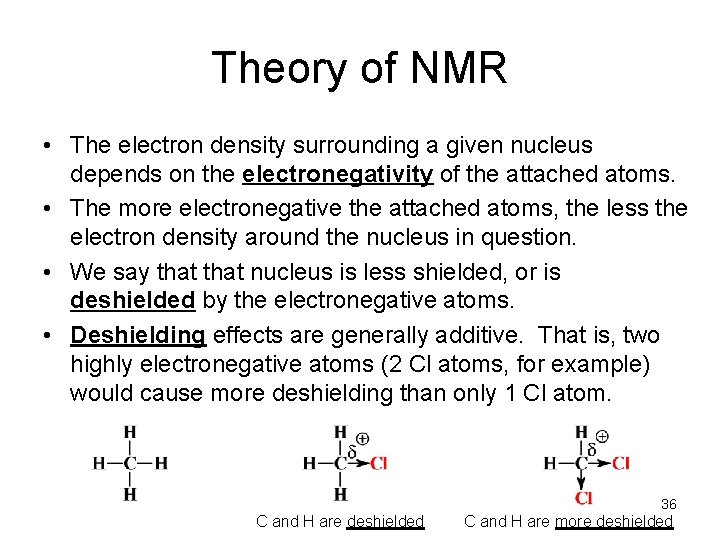
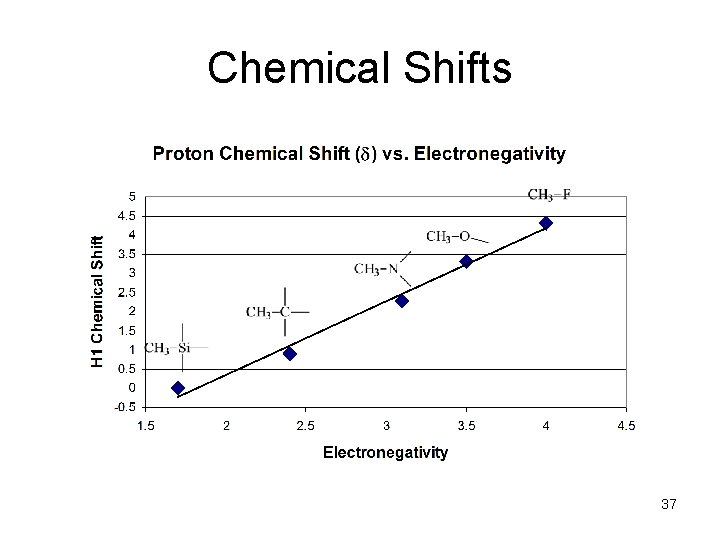
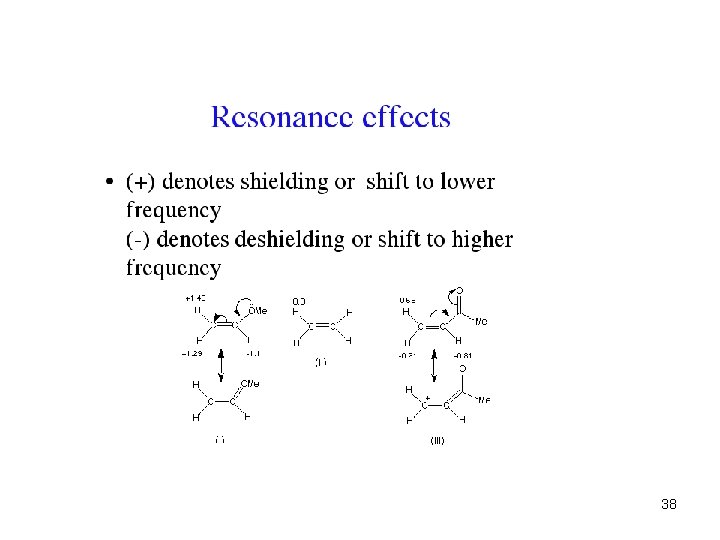
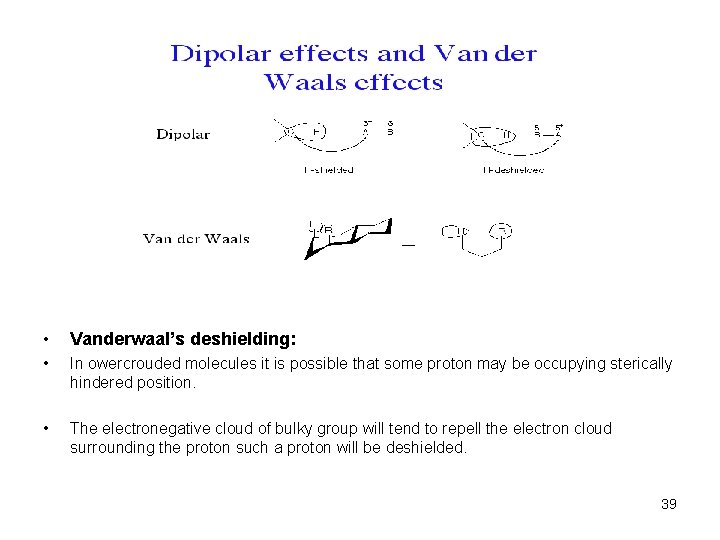
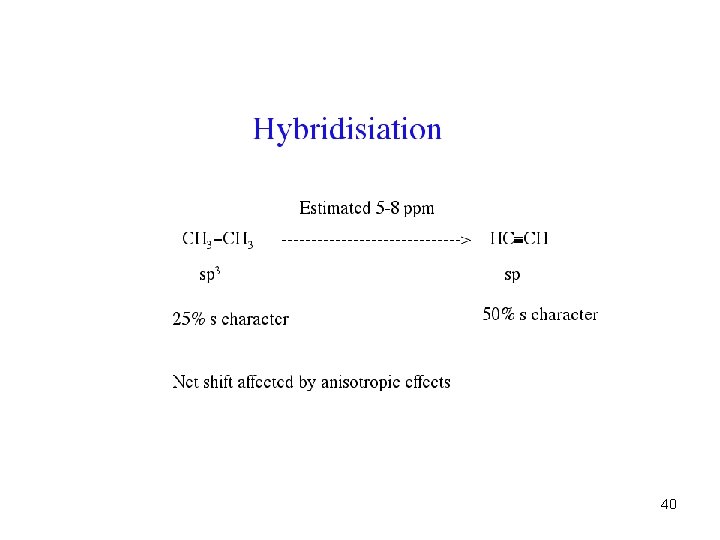
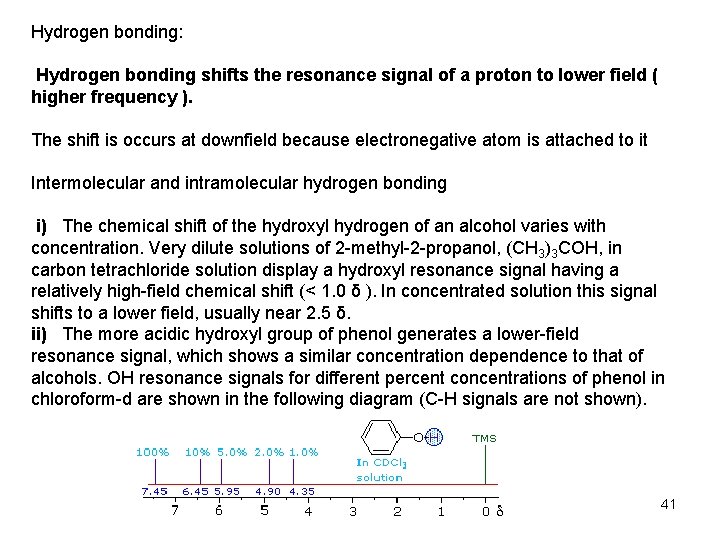
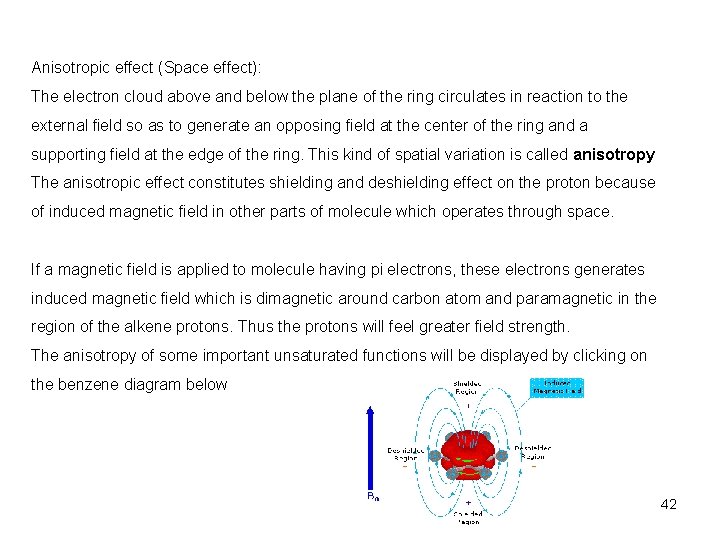
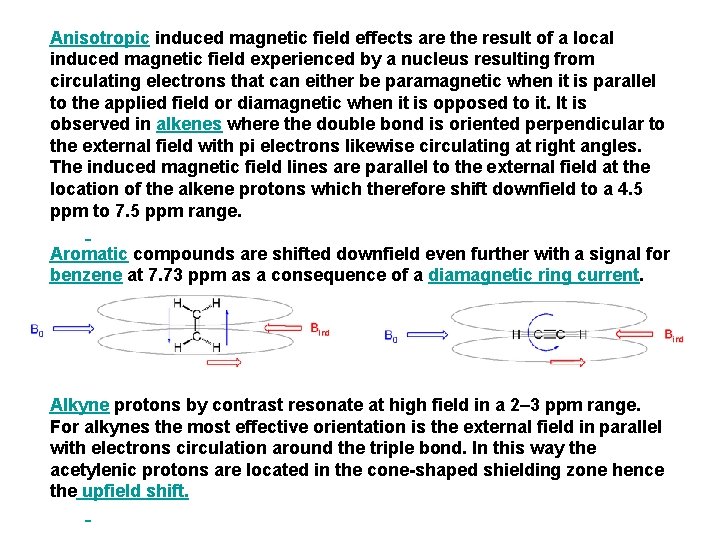
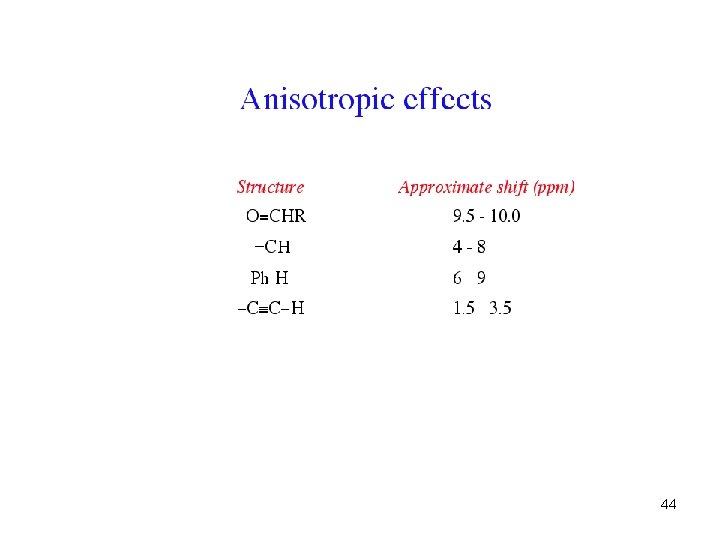
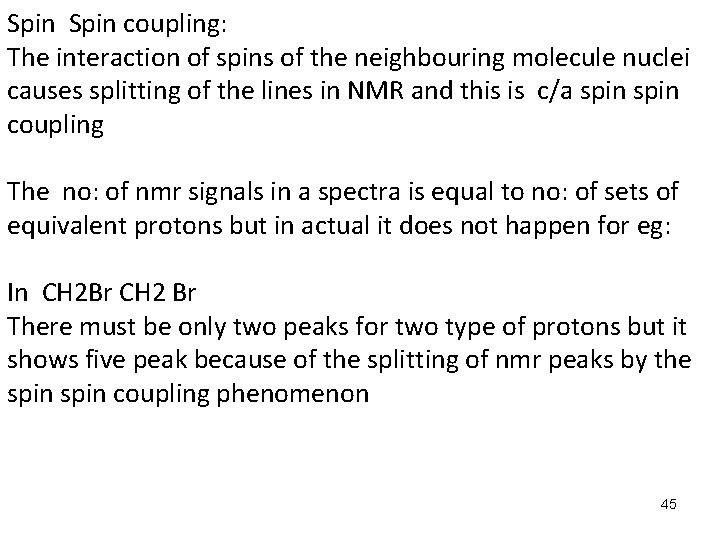
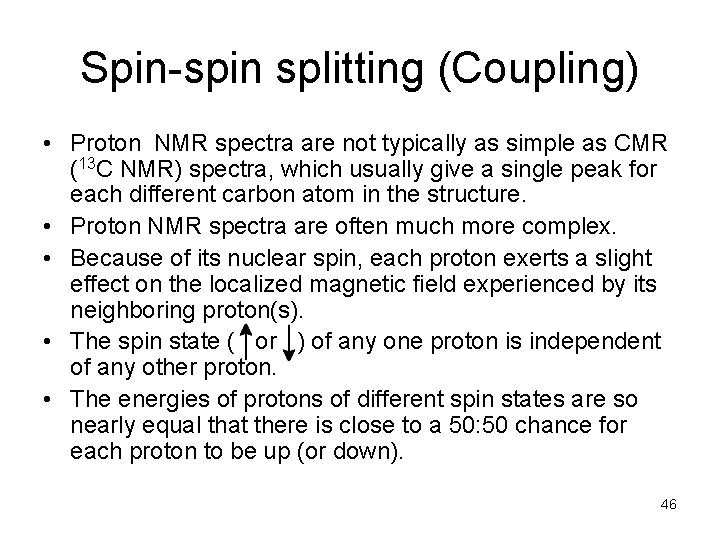
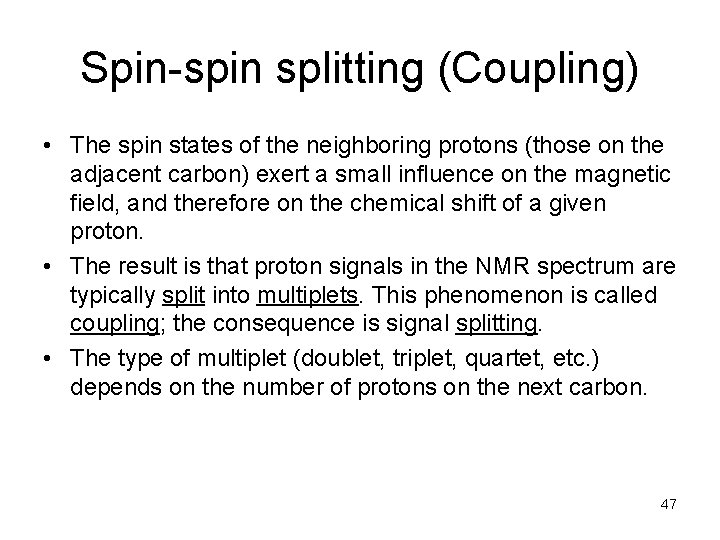
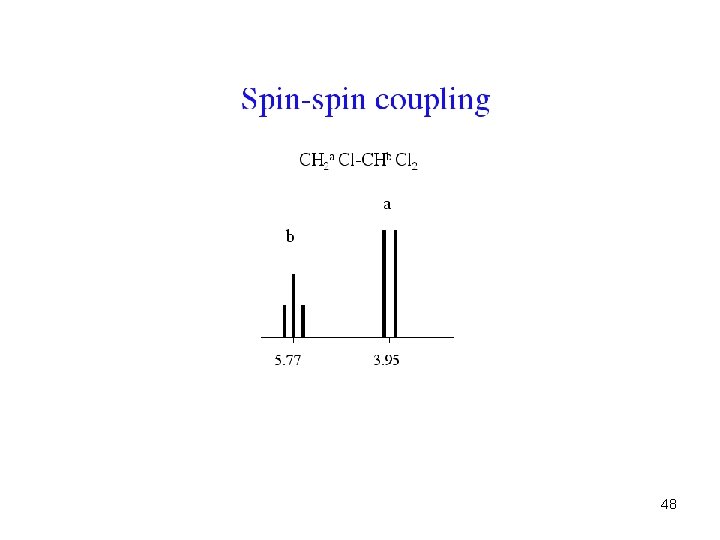
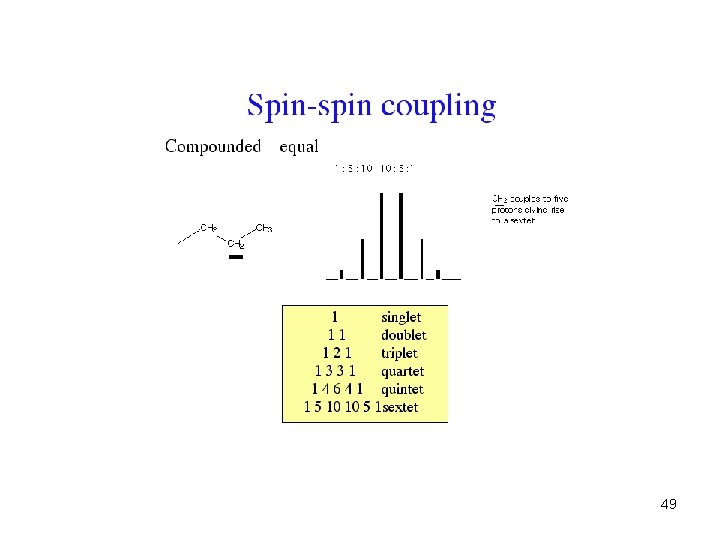
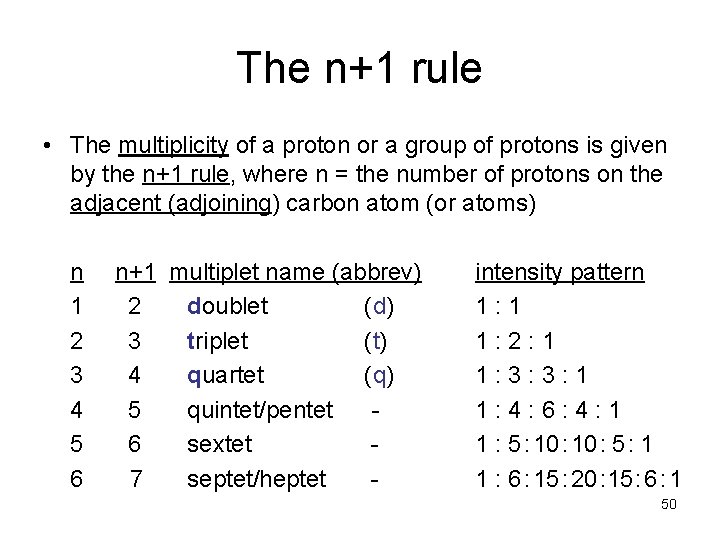
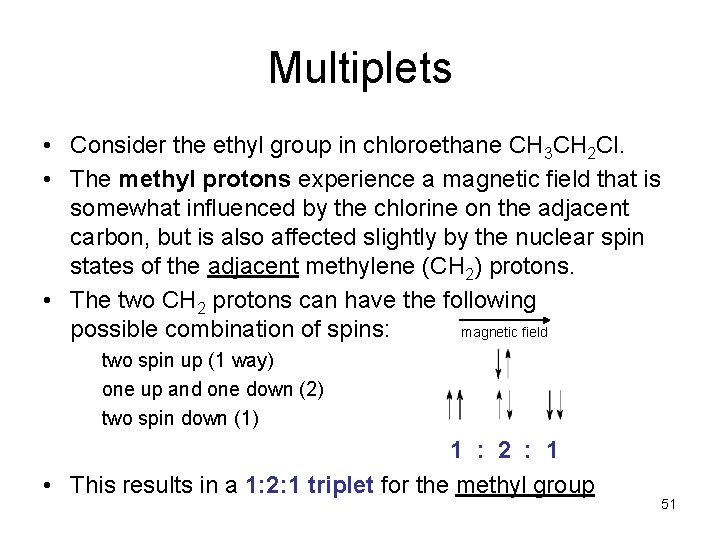
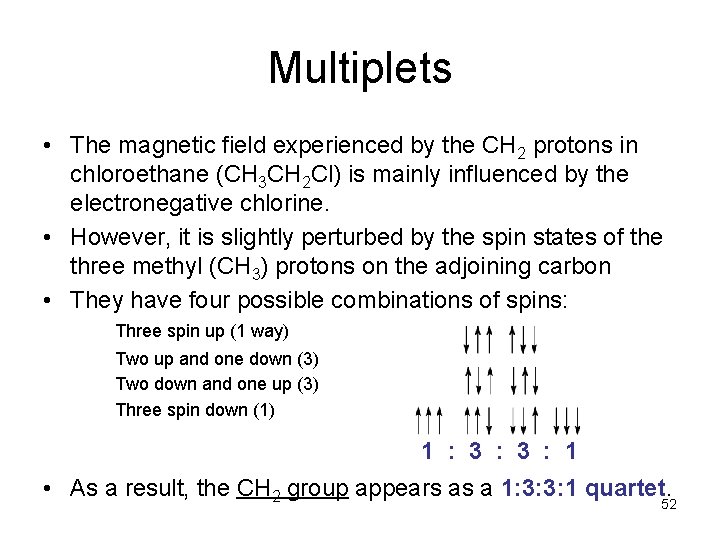
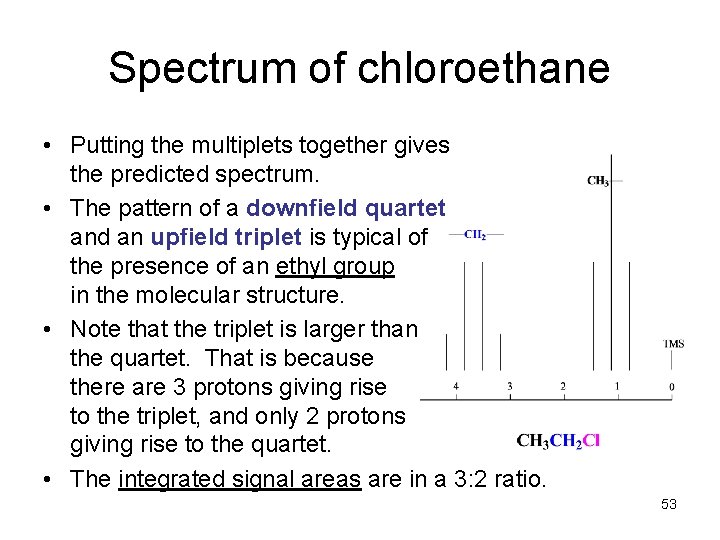
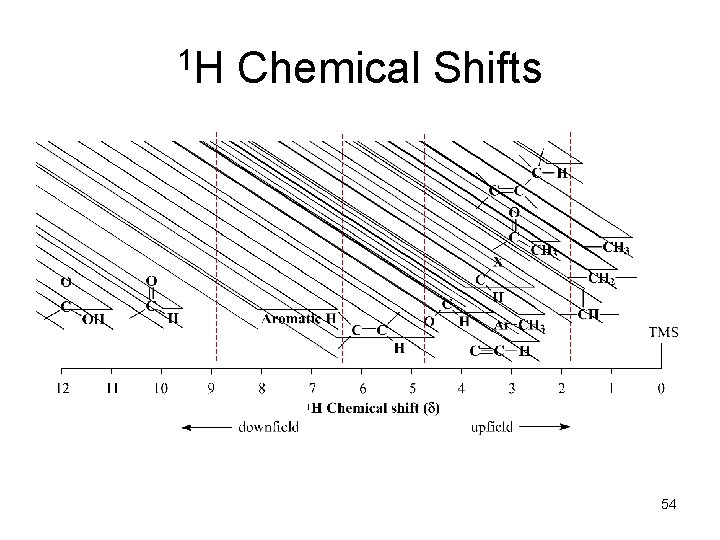
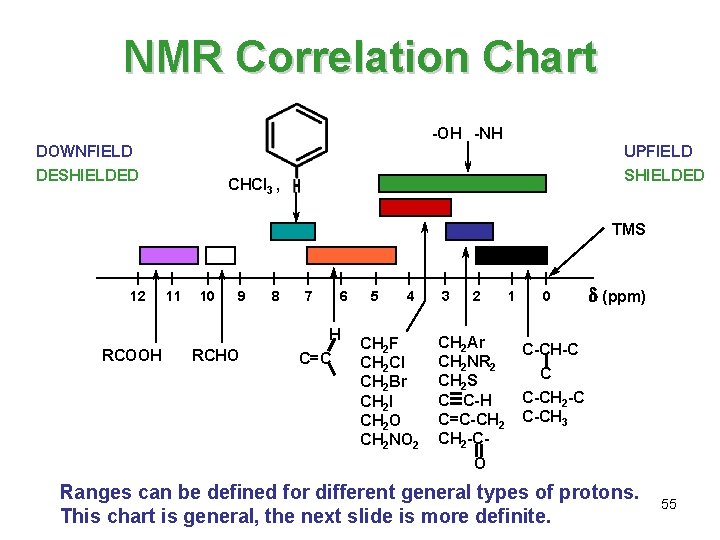
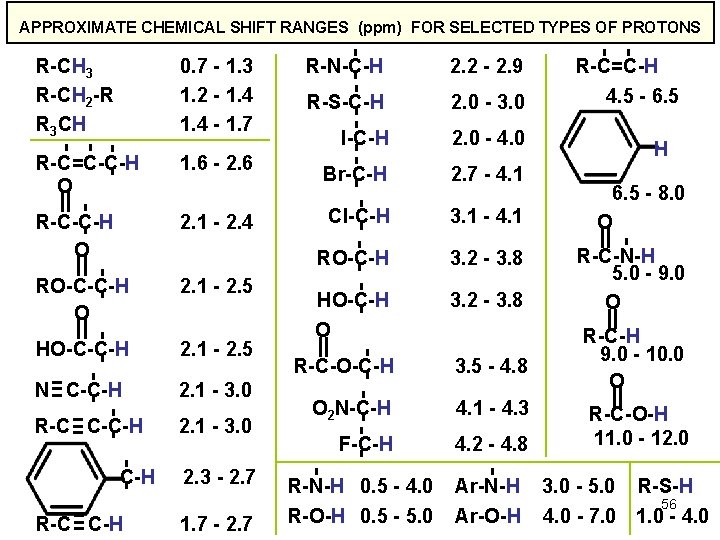
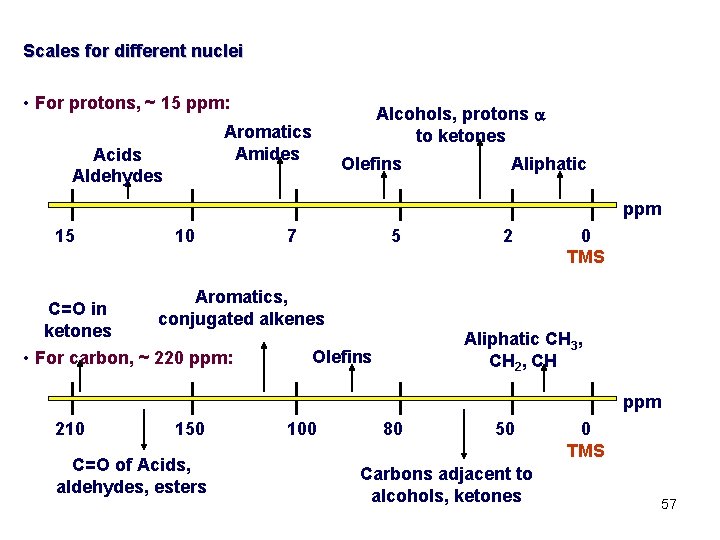
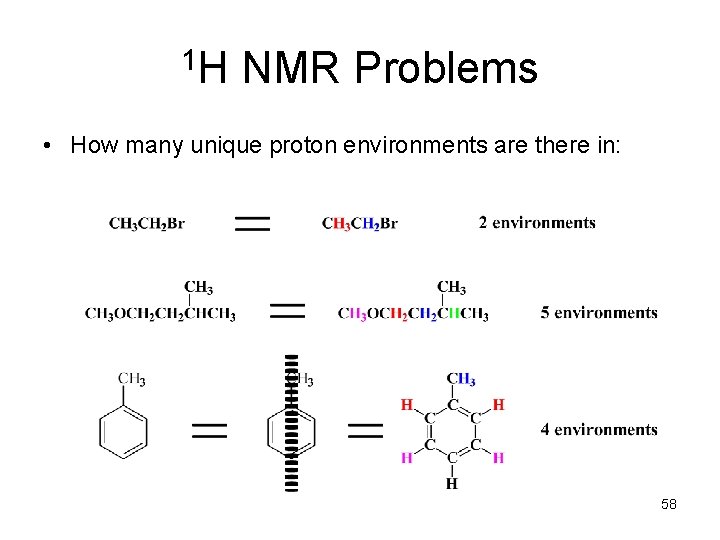
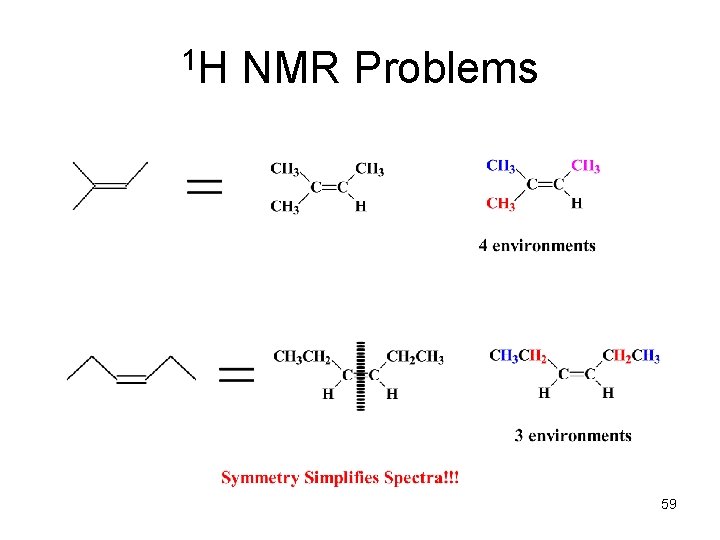
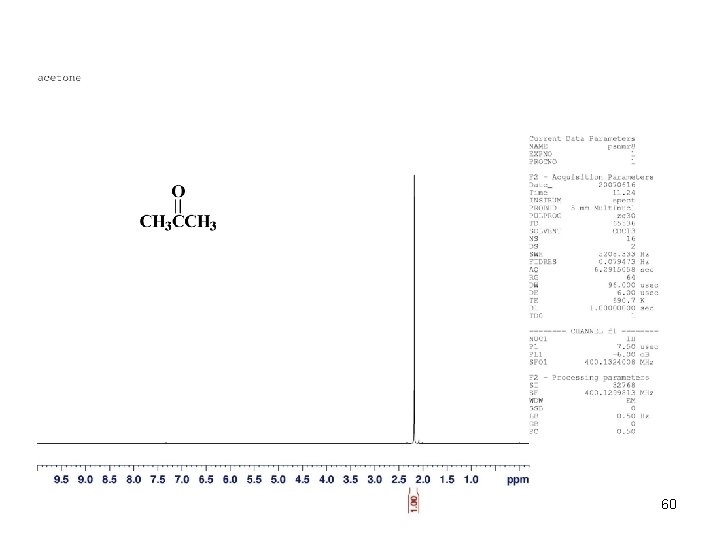
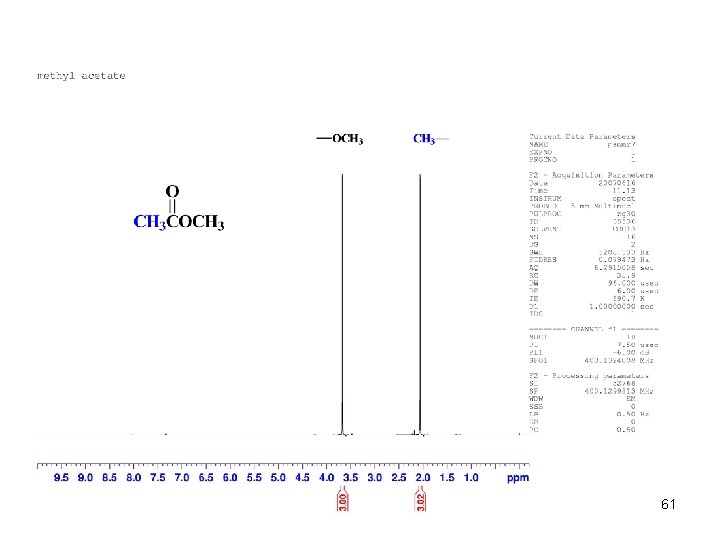
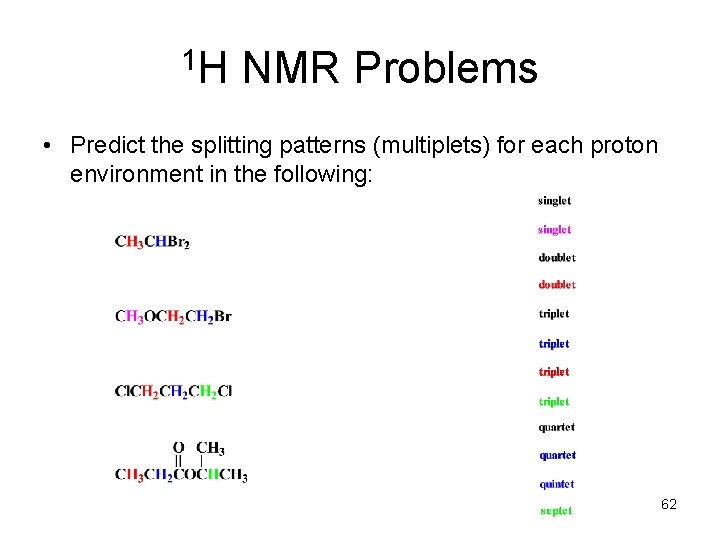
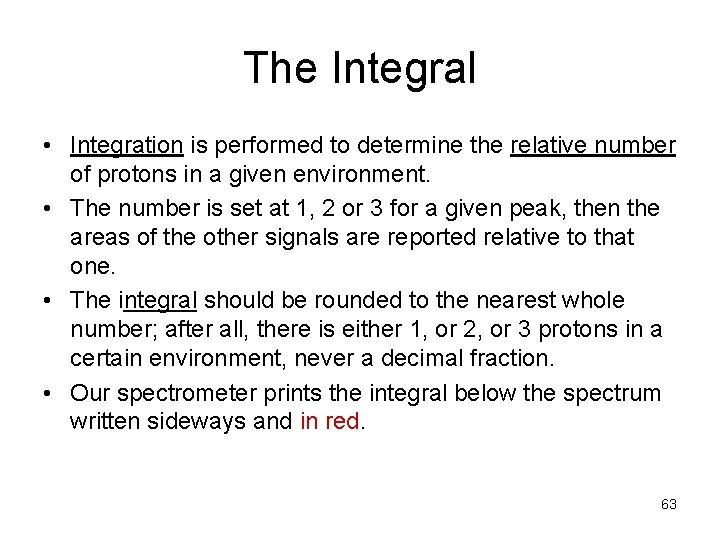
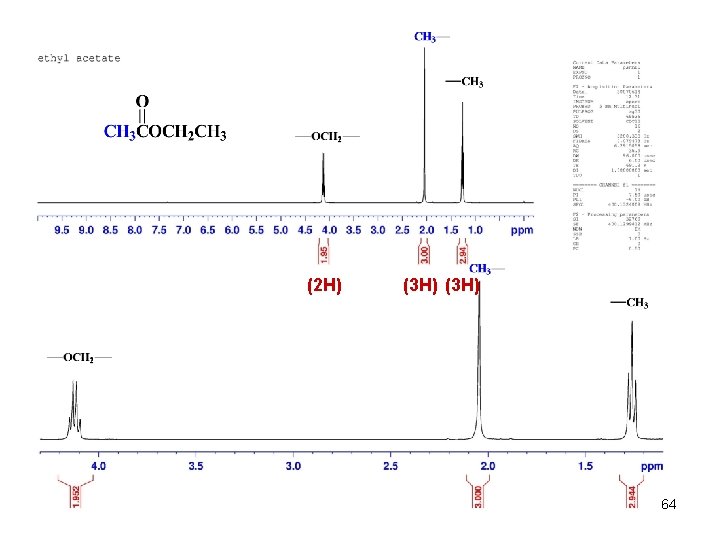
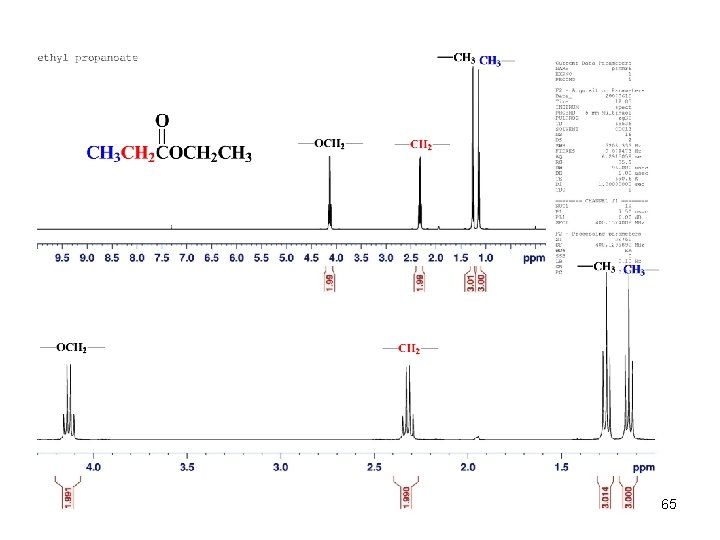
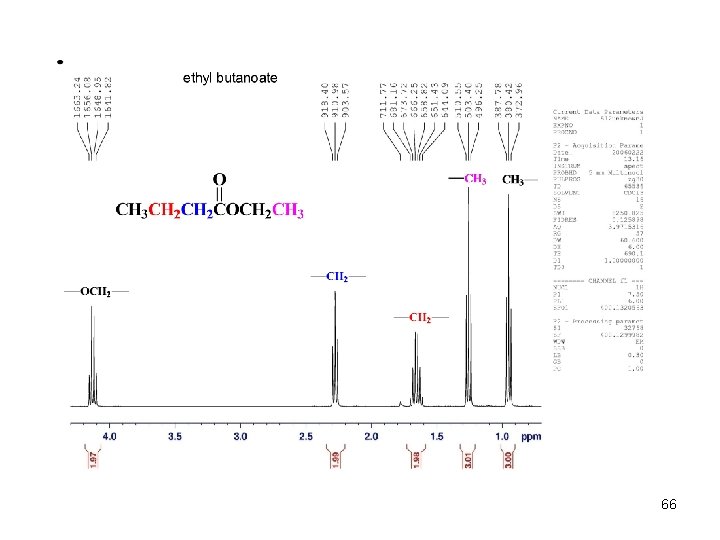
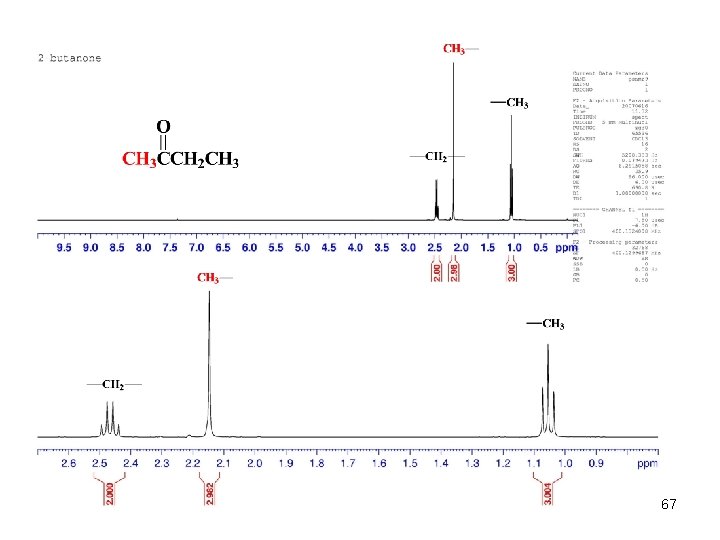
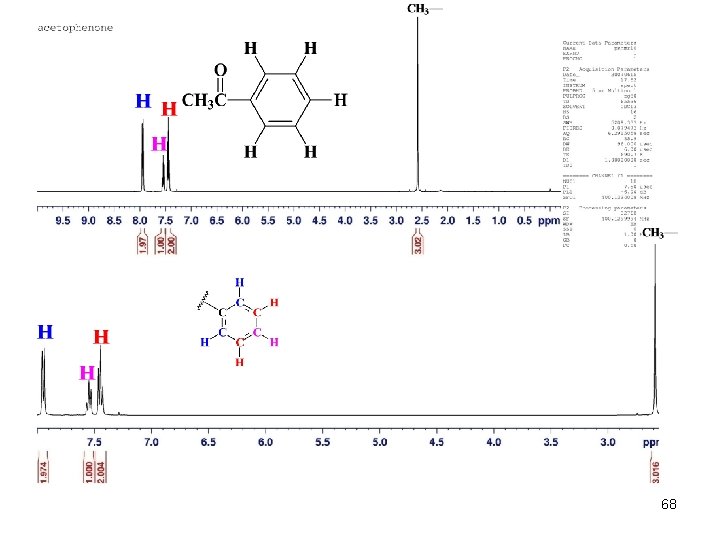
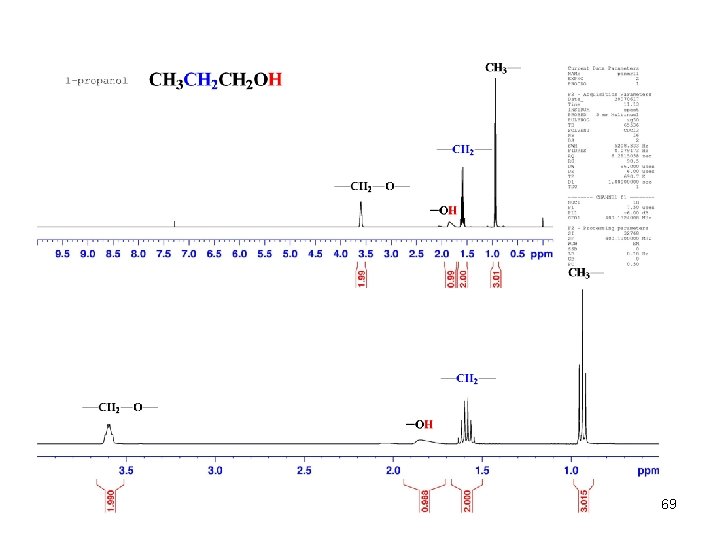
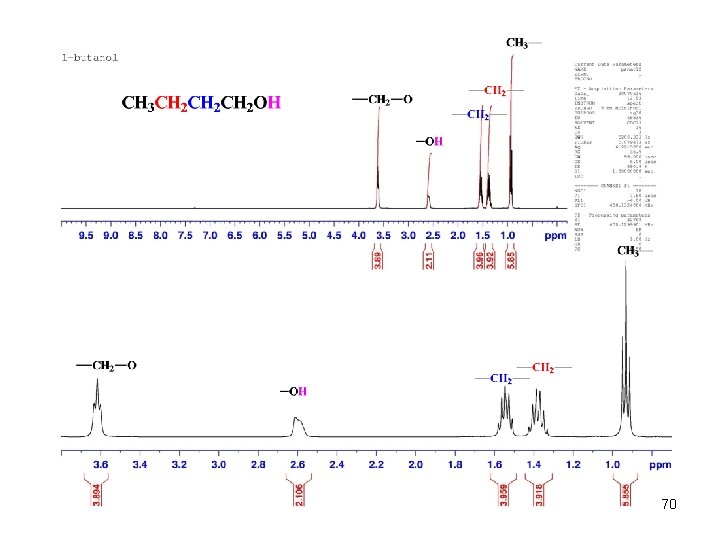
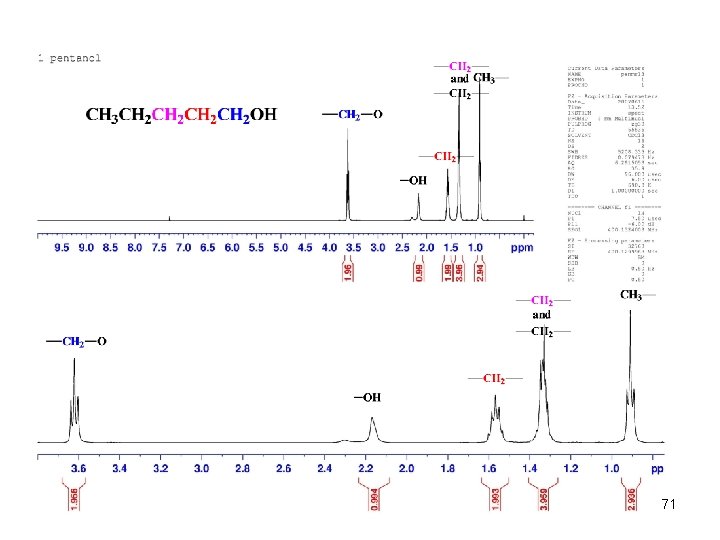
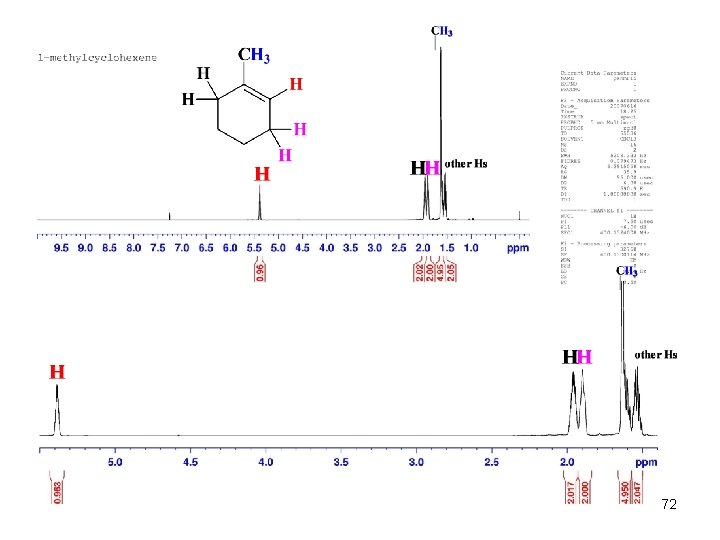
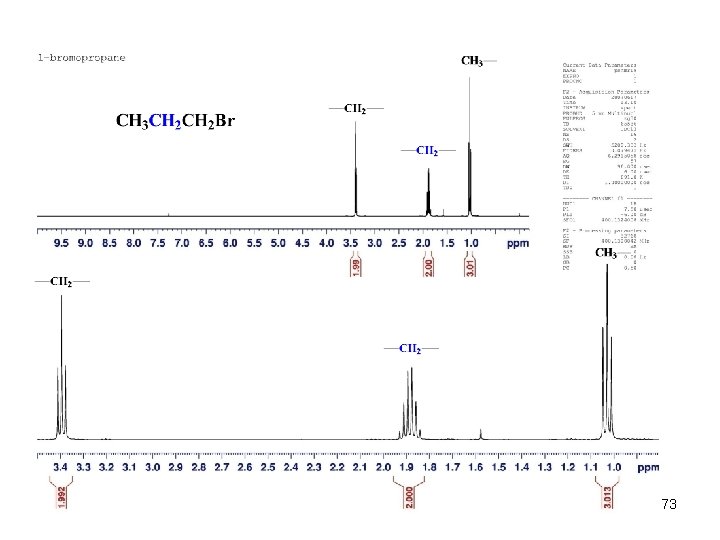
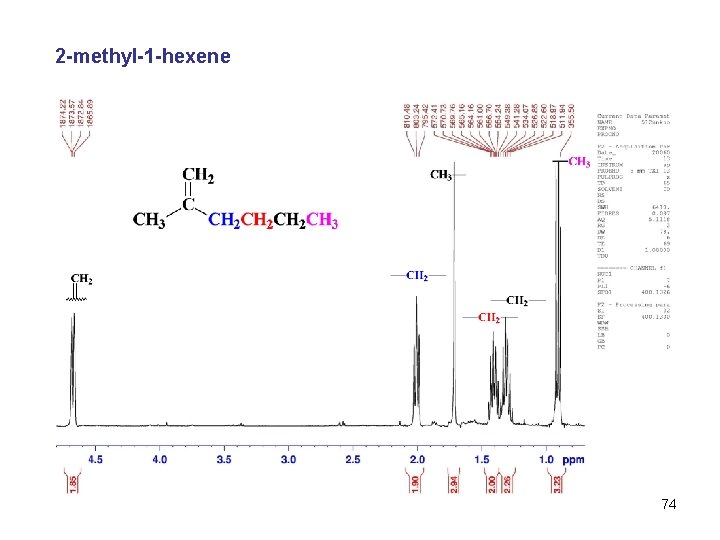
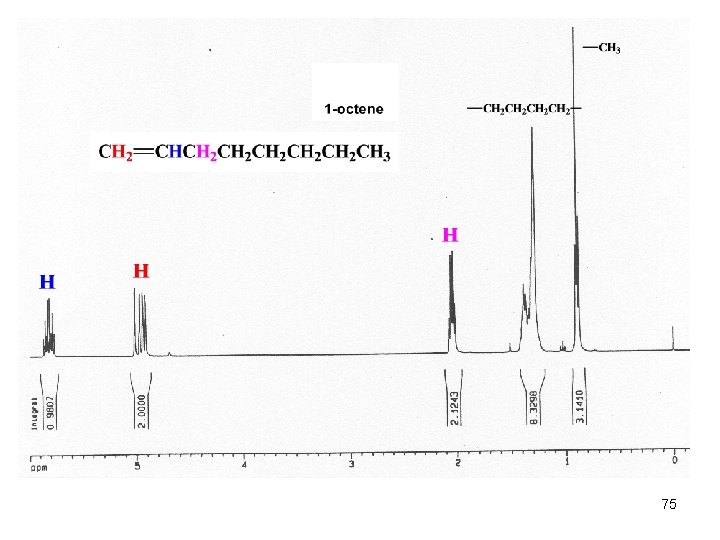
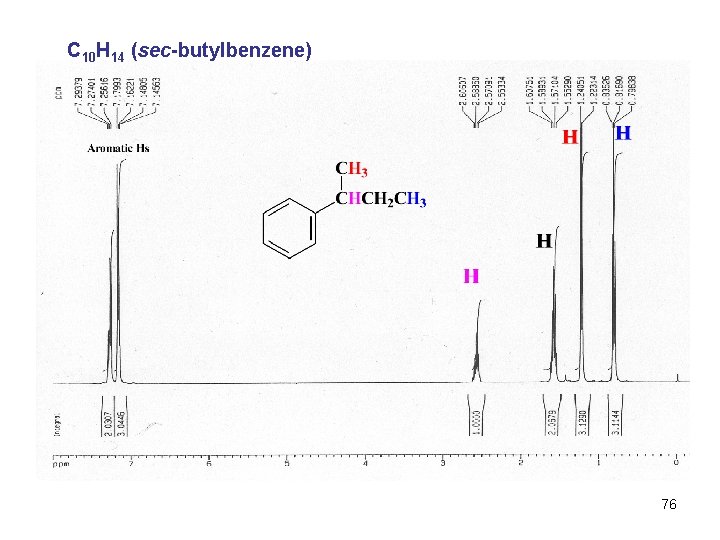
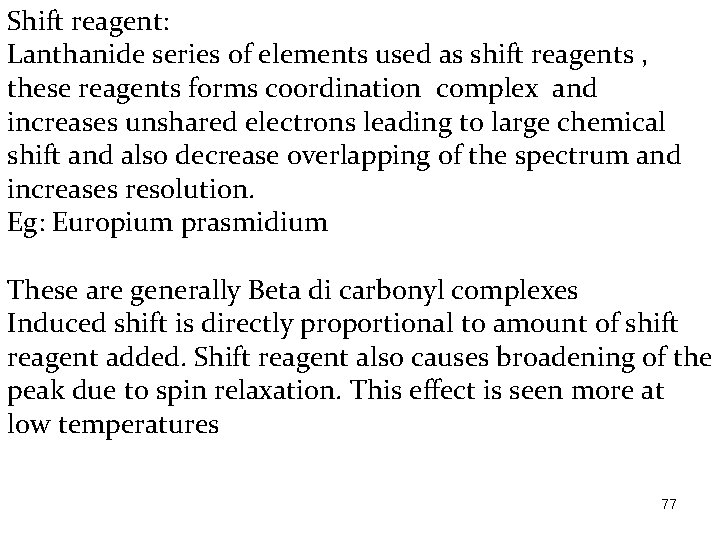
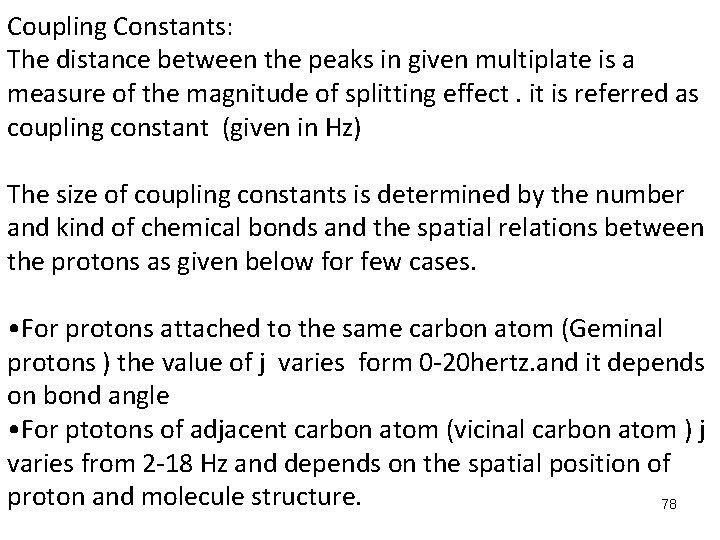
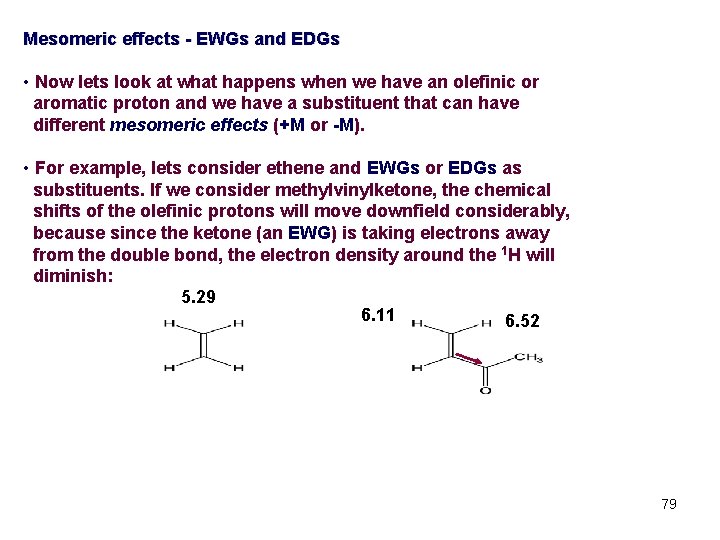
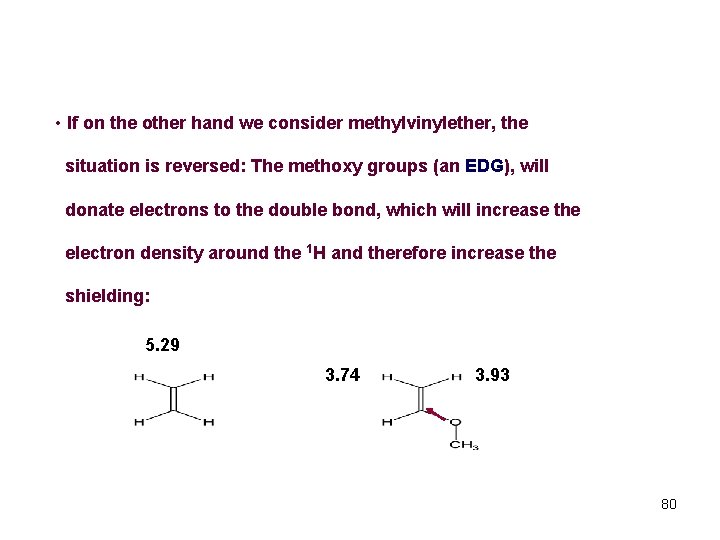
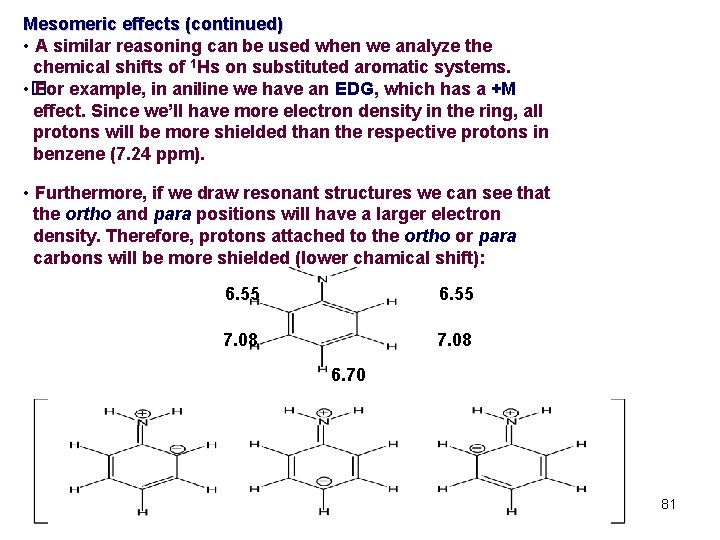
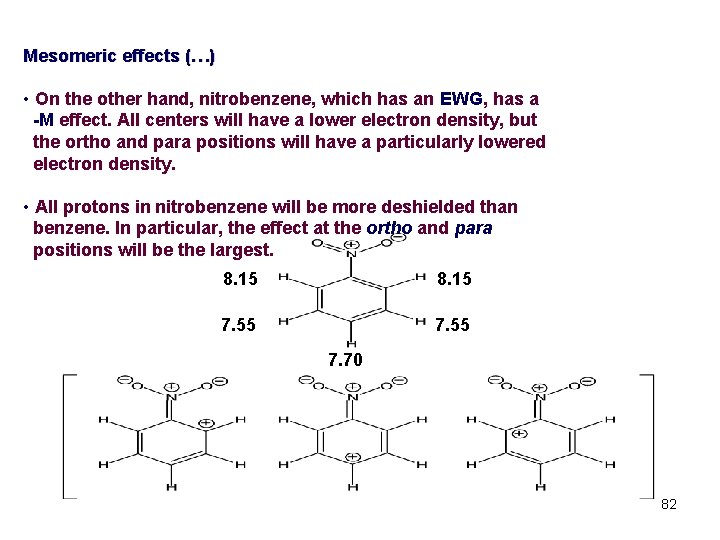
- Slides: 82
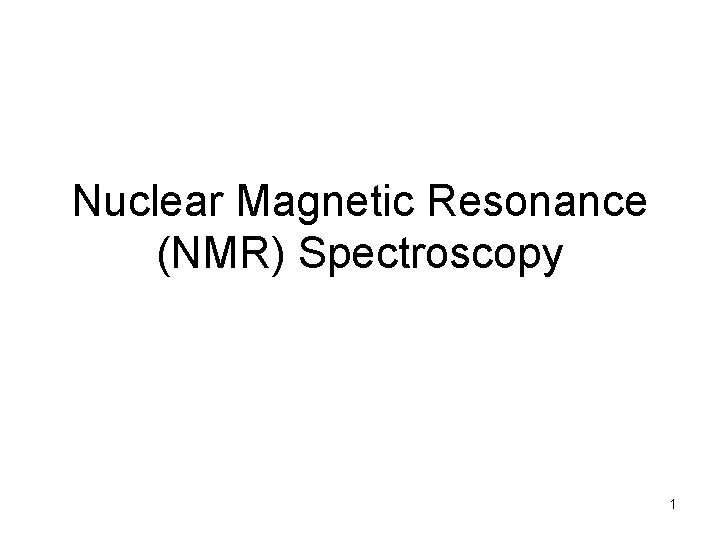
Nuclear Magnetic Resonance (NMR) Spectroscopy 1
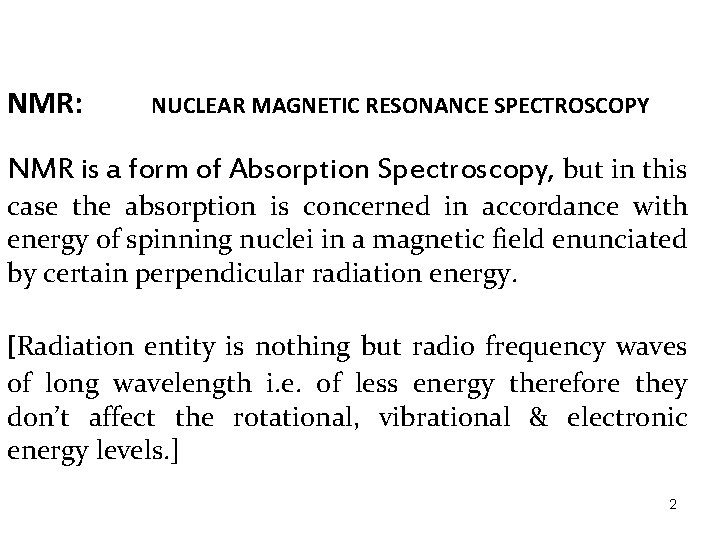
NMR: NUCLEAR MAGNETIC RESONANCE SPECTROSCOPY NMR is a form of Absorption Spectroscopy, but in this case the absorption is concerned in accordance with energy of spinning nuclei in a magnetic field enunciated by certain perpendicular radiation energy. [Radiation entity is nothing but radio frequency waves of long wavelength i. e. of less energy therefore they don’t affect the rotational, vibrational & electronic energy levels. ] 2
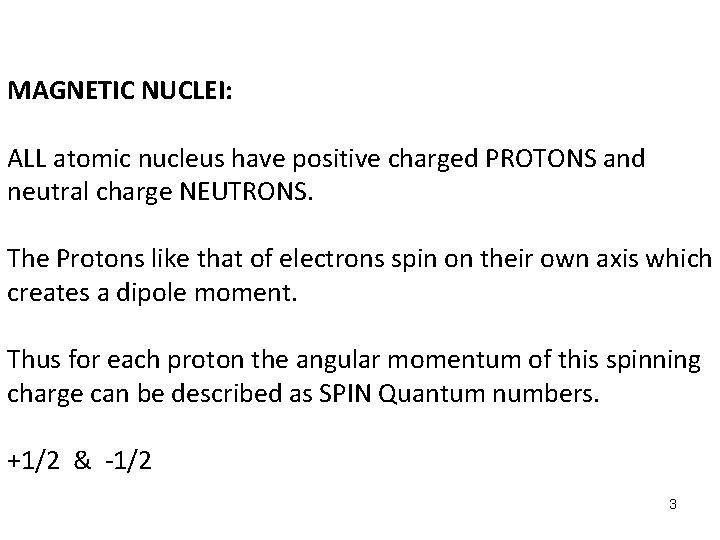
MAGNETIC NUCLEI: ALL atomic nucleus have positive charged PROTONS and neutral charge NEUTRONS. The Protons like that of electrons spin on their own axis which creates a dipole moment. Thus for each proton the angular momentum of this spinning charge can be described as SPIN Quantum numbers. +1/2 & -1/2 3
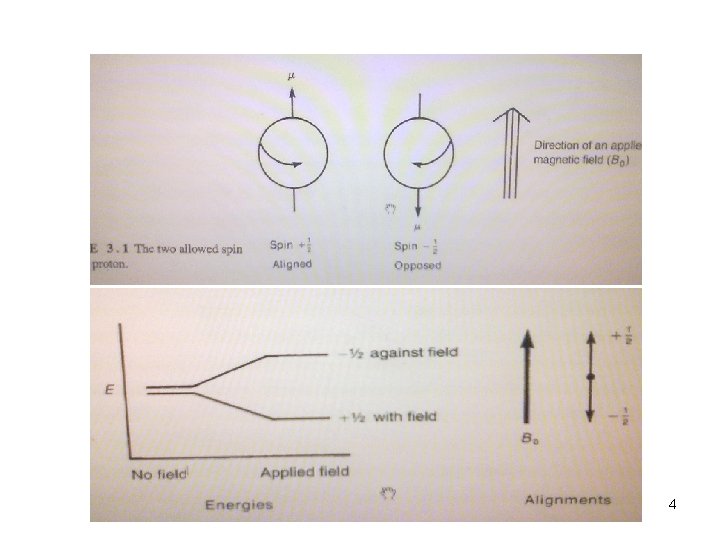
4
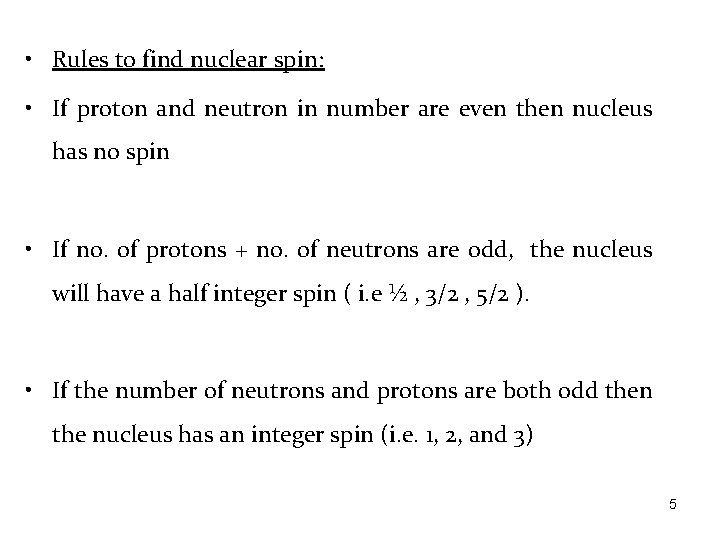
• Rules to find nuclear spin: • If proton and neutron in number are even then nucleus has no spin • If no. of protons + no. of neutrons are odd, the nucleus will have a half integer spin ( i. e ½ , 3/2 , 5/2 ). • If the number of neutrons and protons are both odd then the nucleus has an integer spin (i. e. 1, 2, and 3) 5
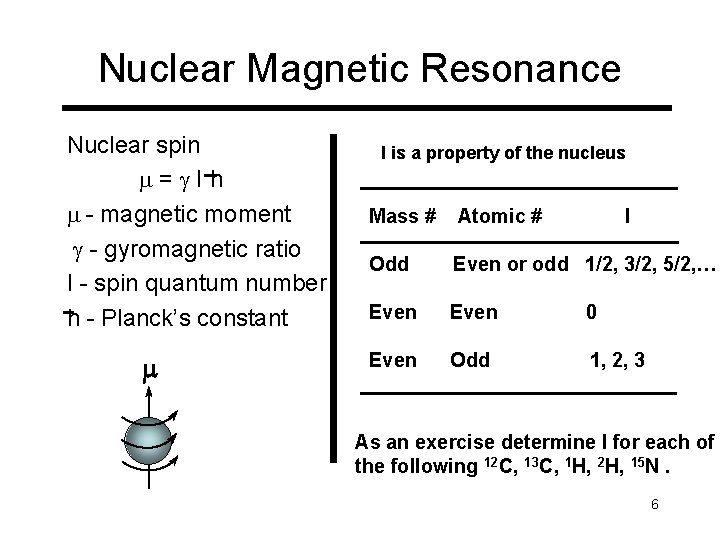
Nuclear Magnetic Resonance Nuclear spin m=g. Ih m - magnetic moment g - gyromagnetic ratio I - spin quantum number h - Planck’s constant m I is a property of the nucleus Mass # Atomic # I Odd Even or odd 1/2, 3/2, 5/2, … Even 0 Even Odd 1, 2, 3 As an exercise determine I for each of the following 12 C, 13 C, 1 H, 2 H, 15 N. 6
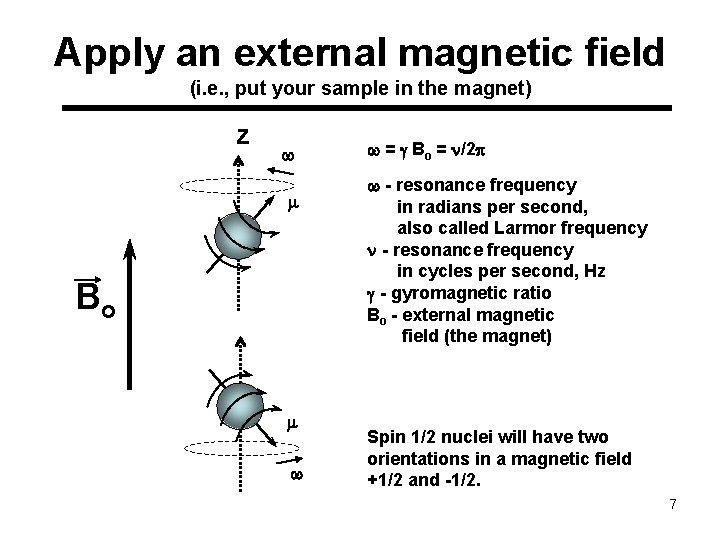
Apply an external magnetic field (i. e. , put your sample in the magnet) z w m Bo m w w = g Bo = n/2 p w - resonance frequency in radians per second, also called Larmor frequency n - resonance frequency in cycles per second, Hz g - gyromagnetic ratio Bo - external magnetic field (the magnet) Spin 1/2 nuclei will have two orientations in a magnetic field +1/2 and -1/2. 7
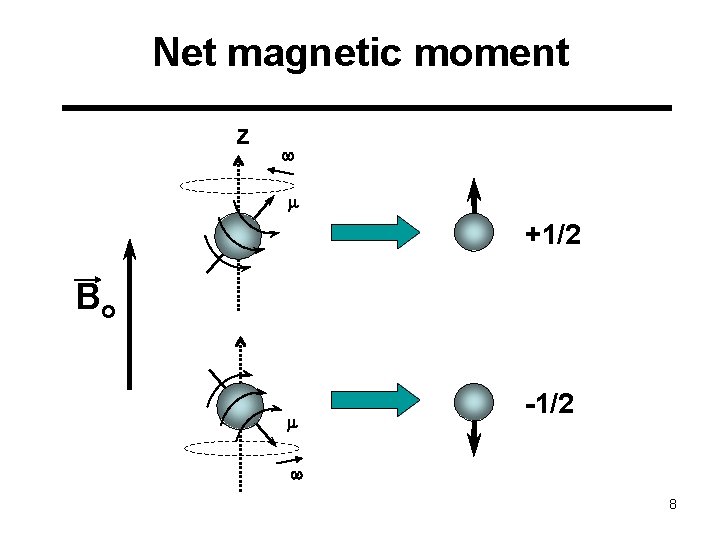
Net magnetic moment z w m +1/2 Bo m -1/2 w 8
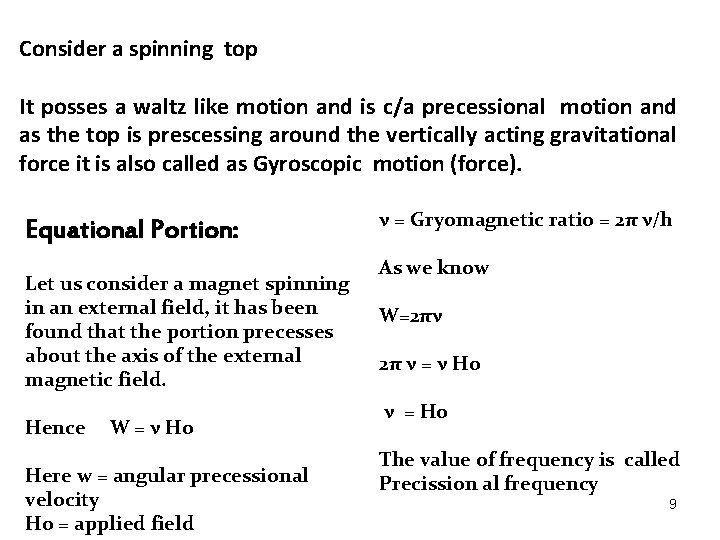
Consider a spinning top It posses a waltz like motion and is c/a precessional motion and as the top is prescessing around the vertically acting gravitational force it is also called as Gyroscopic motion (force). Equational Portion: Let us consider a magnet spinning in an external field, it has been found that the portion precesses about the axis of the external magnetic field. Hence W = ν Ho Here w = angular precessional velocity Ho = applied field ν = Gryomagnetic ratio = 2π ν/h As we know W=2πν 2π ν = ν Ho ν = Ho The value of frequency is called Precission al frequency 9
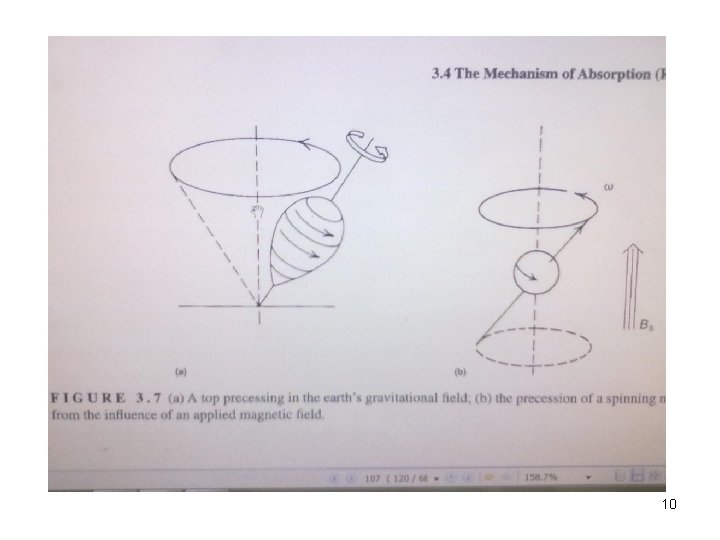
10
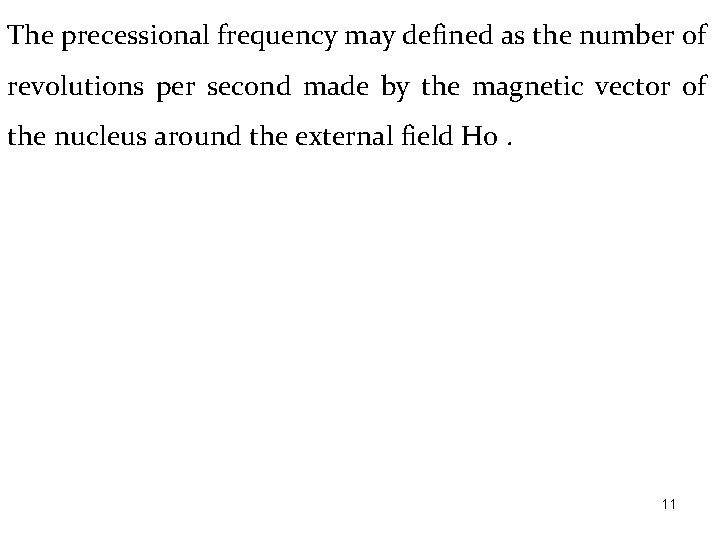
The precessional frequency may defined as the number of revolutions per second made by the magnetic vector of the nucleus around the external field Ho. 11
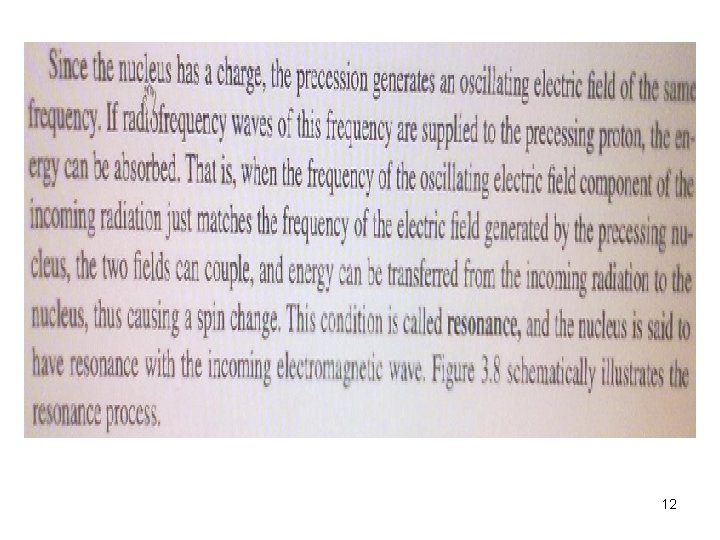
12
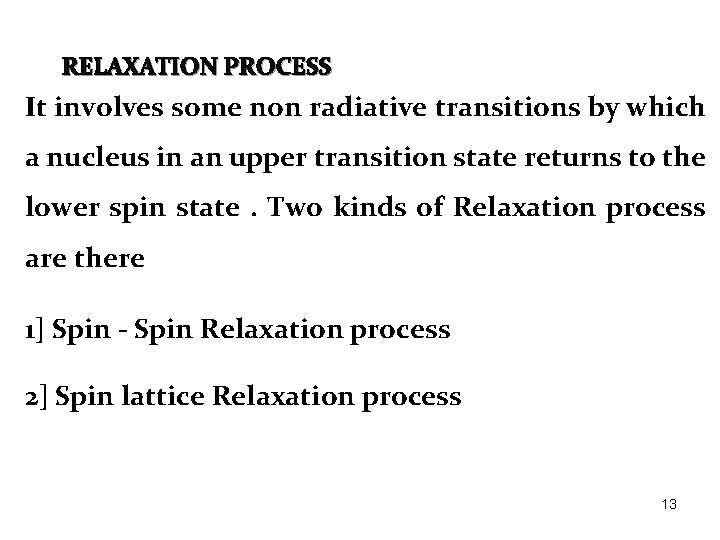
RELAXATION PROCESS It involves some non radiative transitions by which a nucleus in an upper transition state returns to the lower spin state. Two kinds of Relaxation process are there 1] Spin - Spin Relaxation process 2] Spin lattice Relaxation process 13
![1 Spin Spin Relaxation process transverse It is due to mutant exchange of 1] Spin - Spin Relaxation process (transverse) It is due to mutant exchange of](https://slidetodoc.com/presentation_image_h2/76ce4179f00634e0da04039fc9d9f555/image-14.jpg)
1] Spin - Spin Relaxation process (transverse) It is due to mutant exchange of spins by two precessing nuclei which are close to one another. Precessing nucleus Is associated with a magnetic vector component rotating in a plane perpendicular to the field , and if field energy is same as required to induce a transition in neighboring proton then mutual spin exchange occurs. i. e transfer of energy from one nuclei to another. The spread of energy among the nuclei results in line broadening which makes NMR spectra of solids more informative. 14
![2 Spin lattice Relaxation process Longitudinal It involves the transfer of energy from 2 ] Spin lattice Relaxation process (Longitudinal) It involves the transfer of energy from](https://slidetodoc.com/presentation_image_h2/76ce4179f00634e0da04039fc9d9f555/image-15.jpg)
2 ] Spin lattice Relaxation process (Longitudinal) It involves the transfer of energy from the nucleus in its higher energy state to the molecular lattice. The energy transferred to the components of the lattice as the additional transitional, rotational, vibrational & electronic energy levels The total energy of system remains the same. An efficient relaxation process involves a short time & results in the broadening of absorption peak. This mechanism is not effective in solids. 15
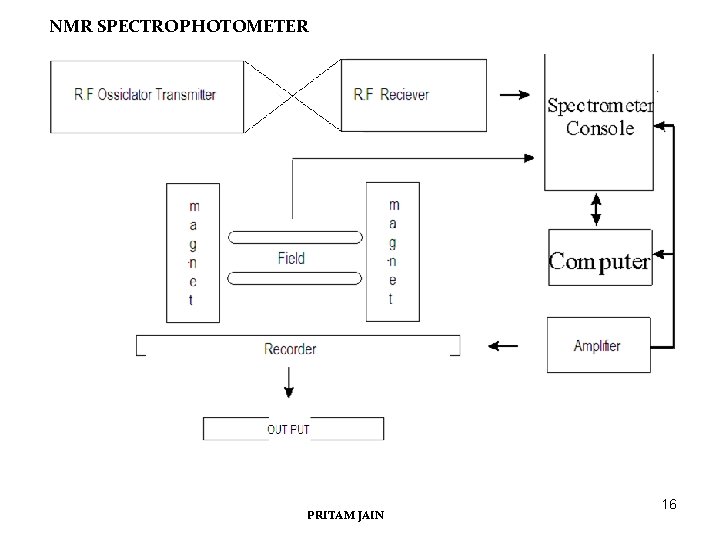
NMR SPECTROPHOTOMETER PRITAM JAIN 16
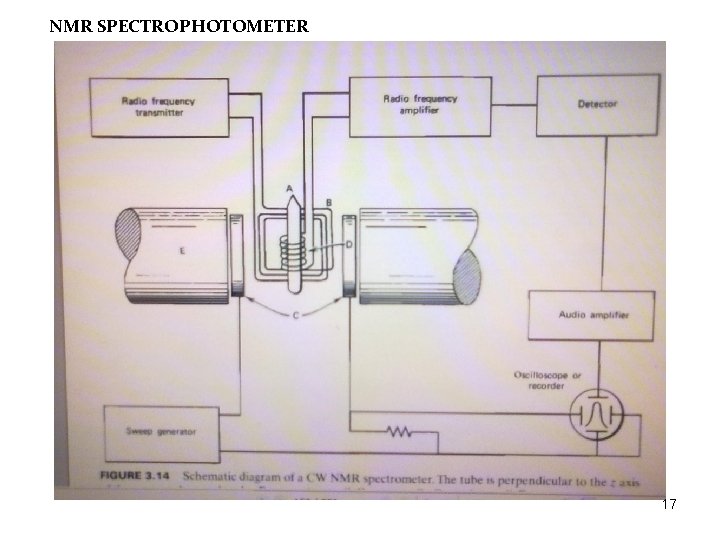
NMR SPECTROPHOTOMETER 17
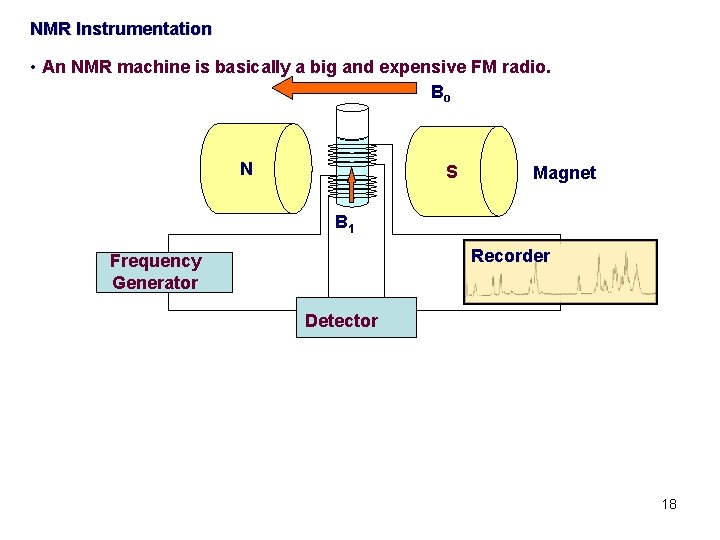
NMR Instrumentation • An NMR machine is basically a big and expensive FM radio. Bo N S Magnet B 1 Recorder Frequency Generator Detector 18
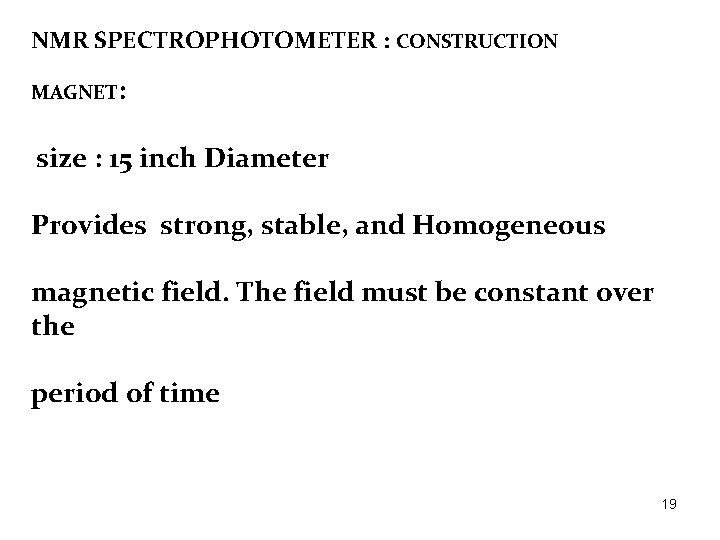
NMR SPECTROPHOTOMETER : CONSTRUCTION MAGNET: size : 15 inch Diameter Provides strong, stable, and Homogeneous magnetic field. The field must be constant over the period of time 19
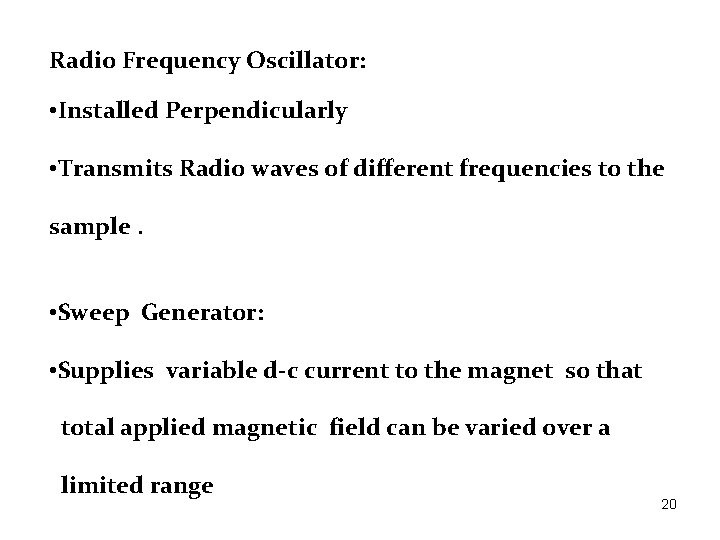
Radio Frequency Oscillator: • Installed Perpendicularly • Transmits Radio waves of different frequencies to the sample. • Sweep Generator: • Supplies variable d-c current to the magnet so that total applied magnetic field can be varied over a limited range 20
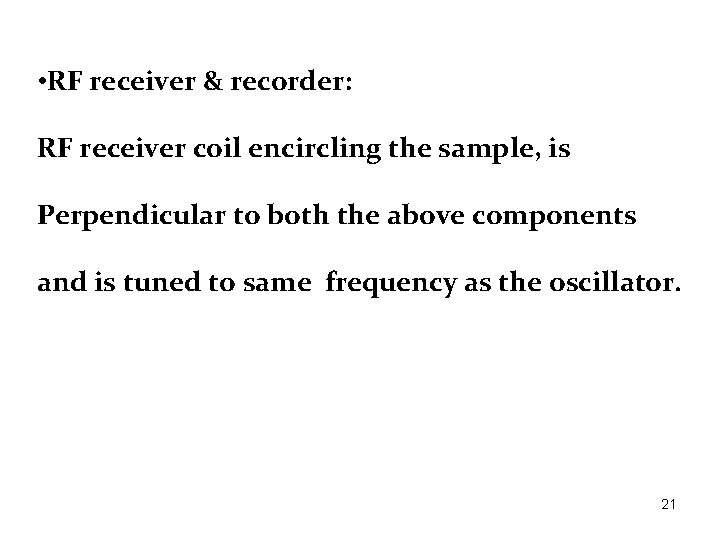
• RF receiver & recorder: RF receiver coil encircling the sample, is Perpendicular to both the above components and is tuned to same frequency as the oscillator. 21
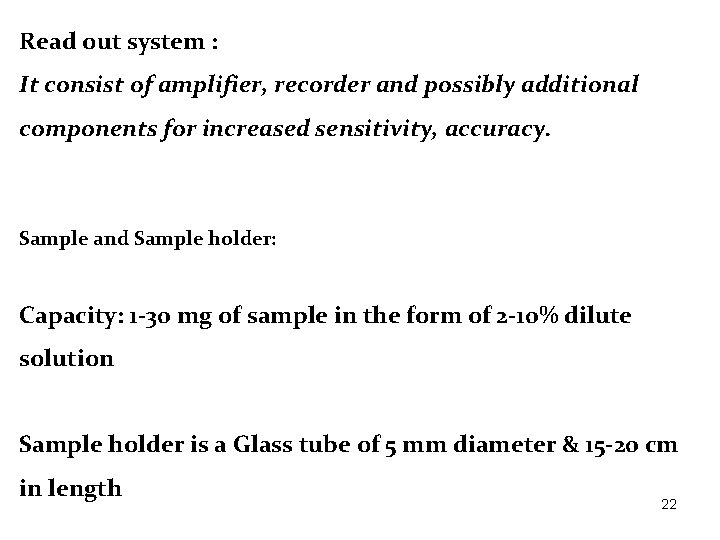
Read out system : It consist of amplifier, recorder and possibly additional components for increased sensitivity, accuracy. Sample and Sample holder: Capacity: 1 -30 mg of sample in the form of 2 -10% dilute solution Sample holder is a Glass tube of 5 mm diameter & 15 -20 cm in length 22
![TMS tetra methyl silane TMS CH 34 Si is used in internal standard because TMS [tetra methyl silane (TMS (CH 3)4 Si)] is used in internal standard because:](https://slidetodoc.com/presentation_image_h2/76ce4179f00634e0da04039fc9d9f555/image-23.jpg)
TMS [tetra methyl silane (TMS (CH 3)4 Si)] is used in internal standard because: • It has twelve equivalent protons which give an intense signal • It is less electronegative • Protons are shielded and thus inert • TMS is volatile and can be removed by evaporation • It is soluble in most organic solvents PRITAM JAIN 23
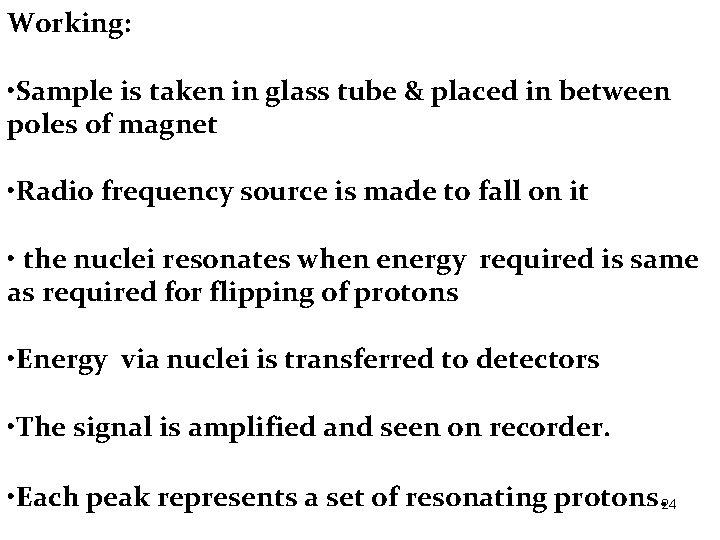
Working: • Sample is taken in glass tube & placed in between poles of magnet • Radio frequency source is made to fall on it • the nuclei resonates when energy required is same as required for flipping of protons • Energy via nuclei is transferred to detectors • The signal is amplified and seen on recorder. • Each peak represents a set of resonating protons. 24
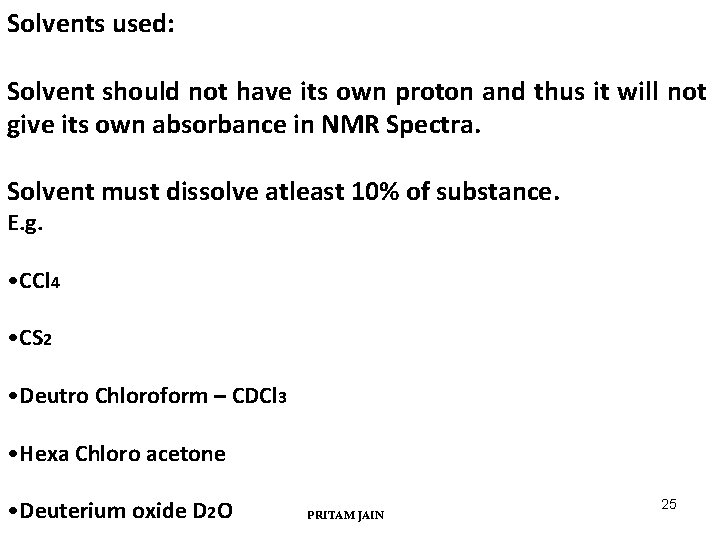
Solvents used: Solvent should not have its own proton and thus it will not give its own absorbance in NMR Spectra. Solvent must dissolve atleast 10% of substance. E. g. • CCl 4 • CS 2 • Deutro Chloroform – CDCl 3 • Hexa Chloro acetone • Deuterium oxide D 2 O PRITAM JAIN 25
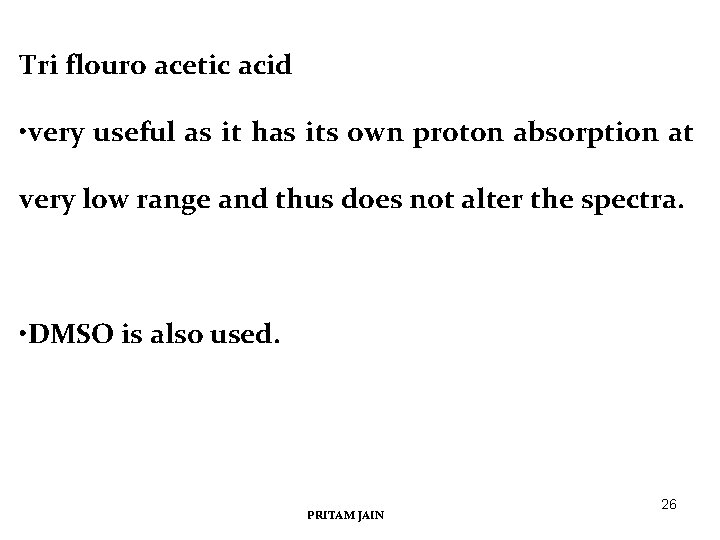
Tri flouro acetic acid • very useful as it has its own proton absorption at very low range and thus does not alter the spectra. • DMSO is also used. PRITAM JAIN 26
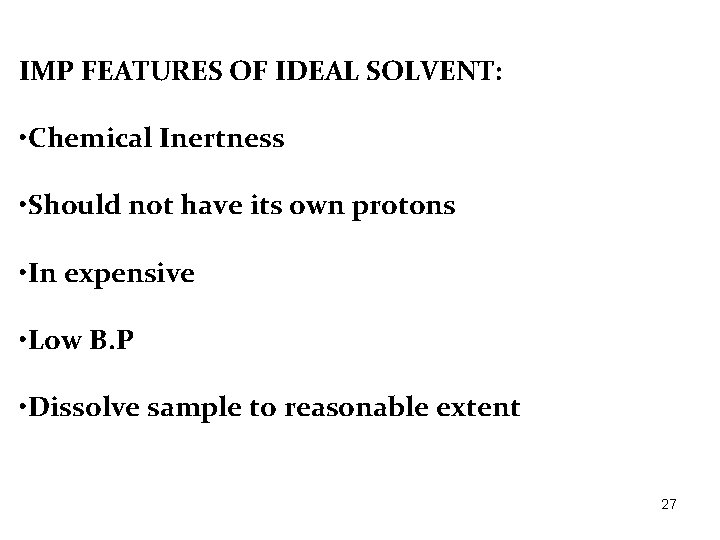
IMP FEATURES OF IDEAL SOLVENT: • Chemical Inertness • Should not have its own protons • In expensive • Low B. P • Dissolve sample to reasonable extent 27
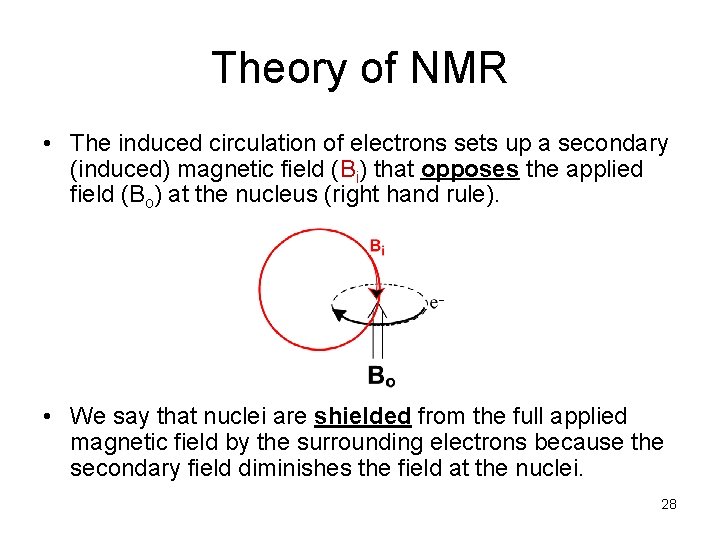
Theory of NMR • The induced circulation of electrons sets up a secondary (induced) magnetic field (Bi) that opposes the applied field (Bo) at the nucleus (right hand rule). • We say that nuclei are shielded from the full applied magnetic field by the surrounding electrons because the secondary field diminishes the field at the nuclei. 28
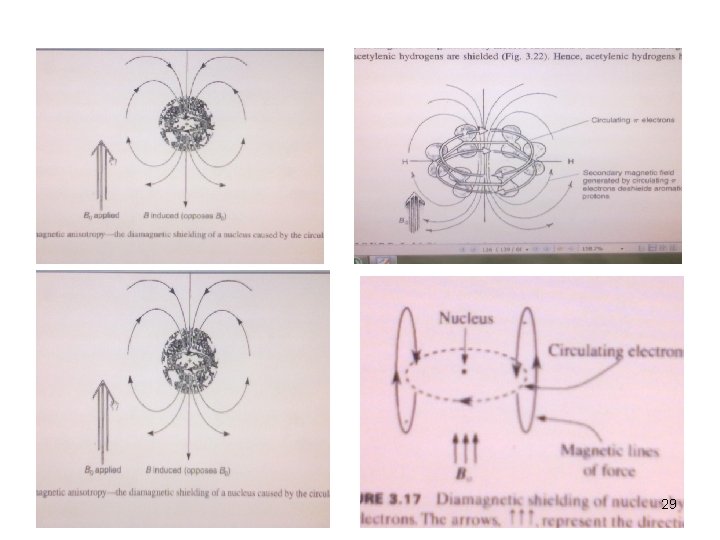
29
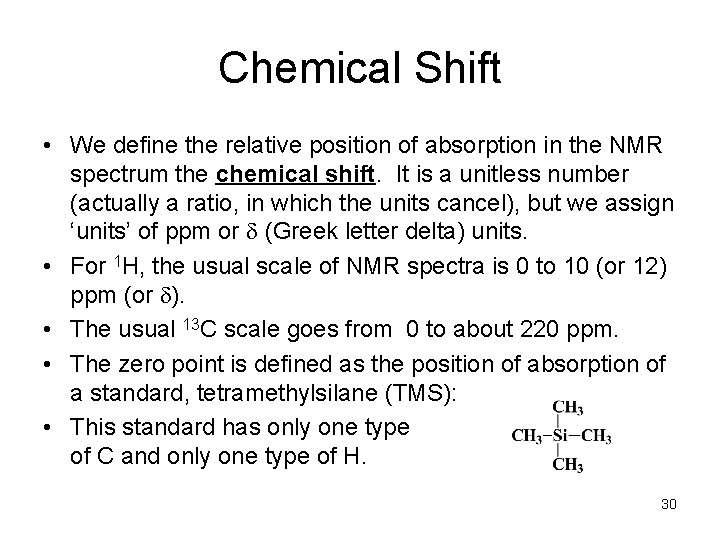
Chemical Shift • We define the relative position of absorption in the NMR spectrum the chemical shift. It is a unitless number (actually a ratio, in which the units cancel), but we assign ‘units’ of ppm or d (Greek letter delta) units. • For 1 H, the usual scale of NMR spectra is 0 to 10 (or 12) ppm (or d). • The usual 13 C scale goes from 0 to about 220 ppm. • The zero point is defined as the position of absorption of a standard, tetramethylsilane (TMS): • This standard has only one type of C and only one type of H. 30
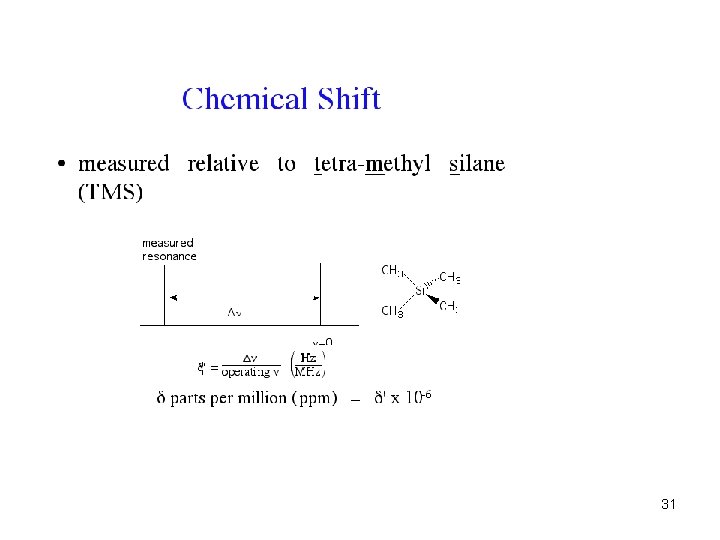
31
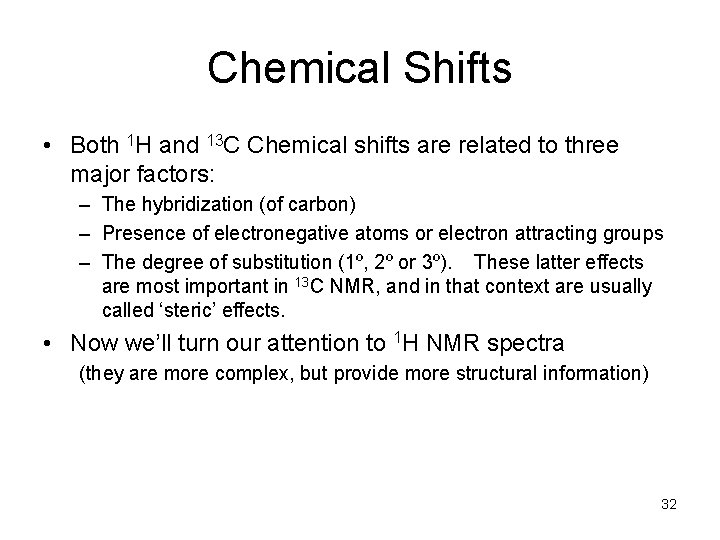
Chemical Shifts • Both 1 H and 13 C Chemical shifts are related to three major factors: – The hybridization (of carbon) – Presence of electronegative atoms or electron attracting groups – The degree of substitution (1º, 2º or 3º). These latter effects are most important in 13 C NMR, and in that context are usually called ‘steric’ effects. • Now we’ll turn our attention to 1 H NMR spectra (they are more complex, but provide more structural information) 32
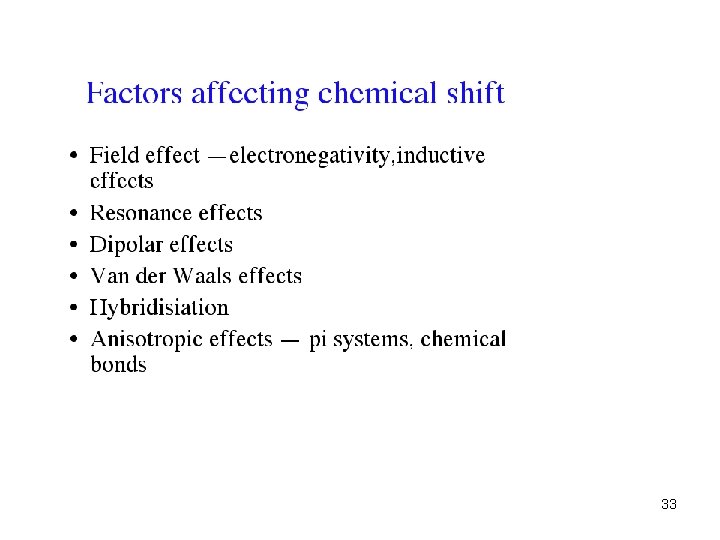
33
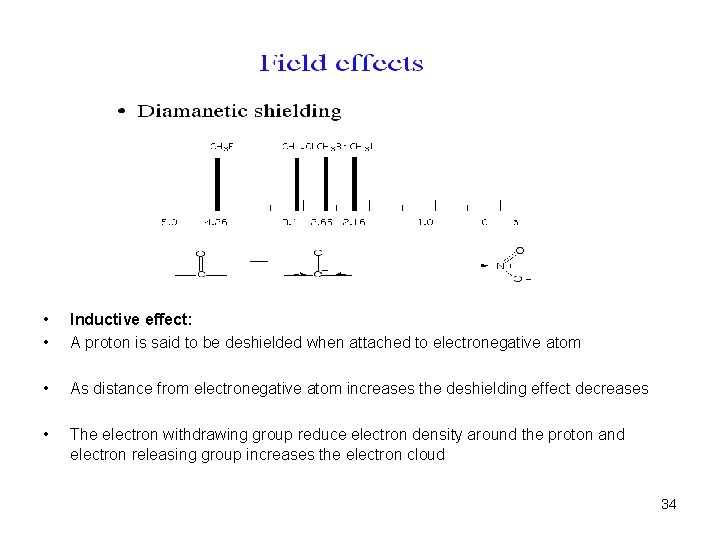
• • Inductive effect: A proton is said to be deshielded when attached to electronegative atom • As distance from electronegative atom increases the deshielding effect decreases • The electron withdrawing group reduce electron density around the proton and electron releasing group increases the electron cloud 34
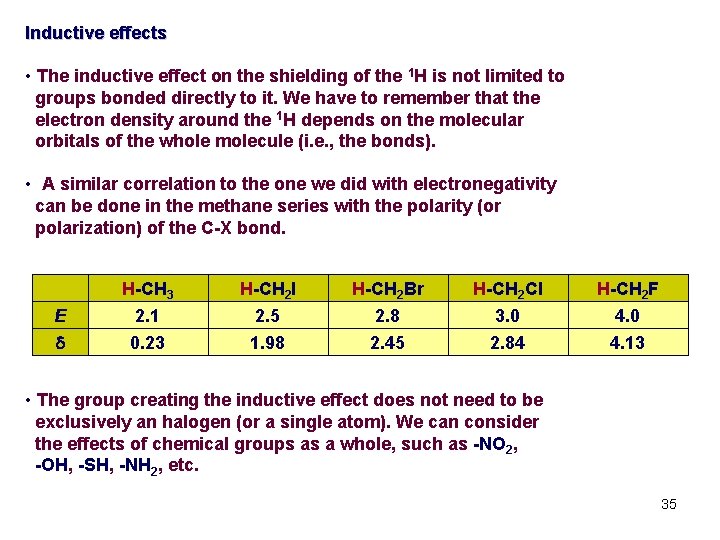
Inductive effects • The inductive effect on the shielding of the 1 H is not limited to groups bonded directly to it. We have to remember that the electron density around the 1 H depends on the molecular orbitals of the whole molecule (i. e. , the bonds). • A similar correlation to the one we did with electronegativity can be done in the methane series with the polarity (or polarization) of the C-X bond. E d H-CH 3 2. 1 0. 23 H-CH 2 I 2. 5 1. 98 H-CH 2 Br 2. 8 2. 45 H-CH 2 Cl 3. 0 2. 84 H-CH 2 F 4. 0 4. 13 • The group creating the inductive effect does not need to be exclusively an halogen (or a single atom). We can consider the effects of chemical groups as a whole, such as -NO 2, -OH, -SH, -NH 2, etc. 35
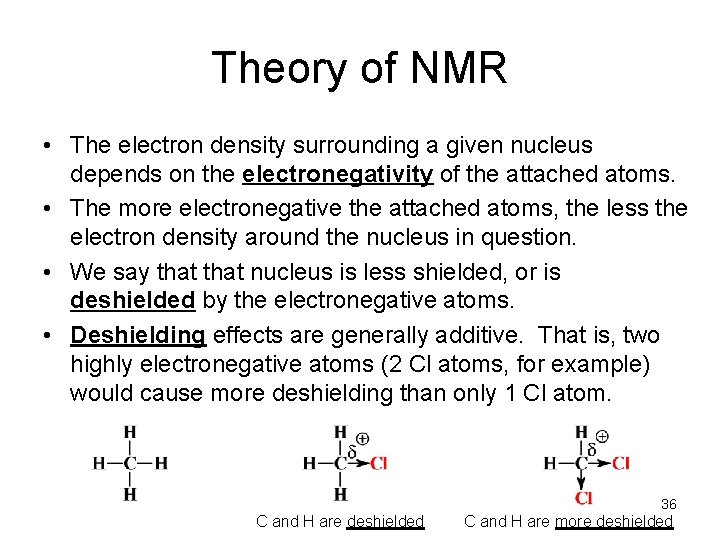
Theory of NMR • The electron density surrounding a given nucleus depends on the electronegativity of the attached atoms. • The more electronegative the attached atoms, the less the electron density around the nucleus in question. • We say that nucleus is less shielded, or is deshielded by the electronegative atoms. • Deshielding effects are generally additive. That is, two highly electronegative atoms (2 Cl atoms, for example) would cause more deshielding than only 1 Cl atom. 36 C and H are deshielded C and H are more deshielded
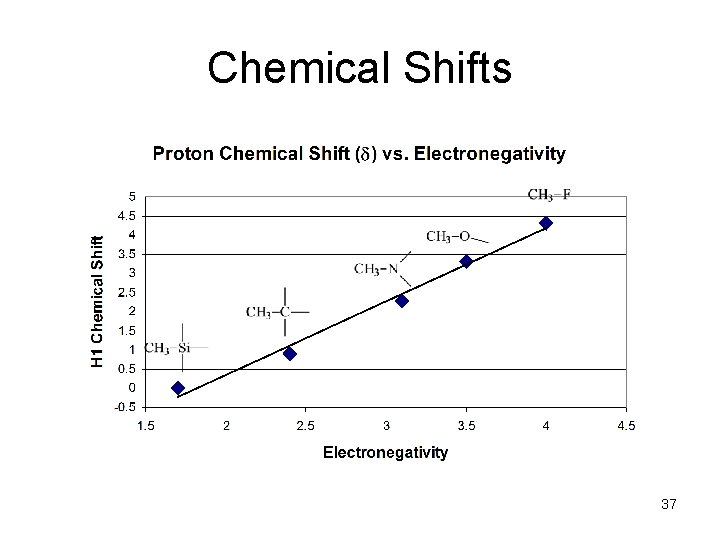
Chemical Shifts 37
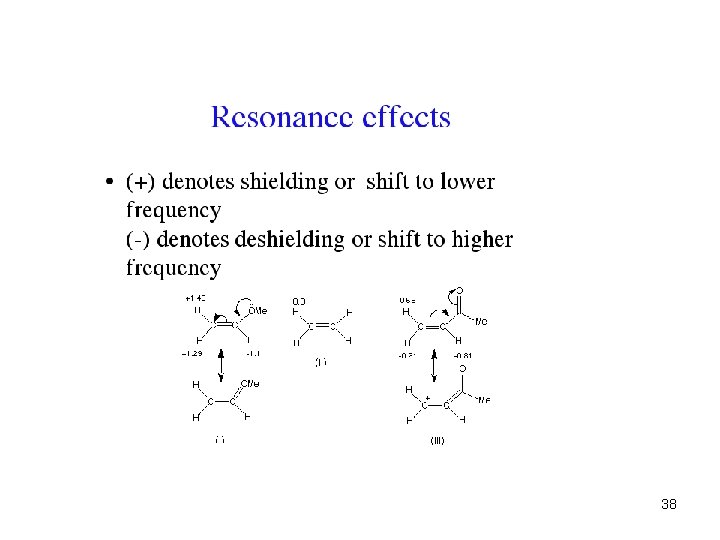
38
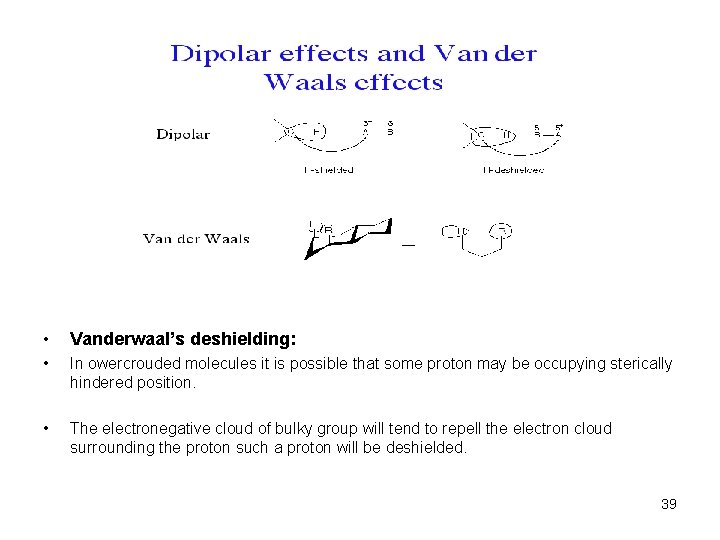
• Vanderwaal’s deshielding: • In owercrouded molecules it is possible that some proton may be occupying sterically hindered position. • The electronegative cloud of bulky group will tend to repell the electron cloud surrounding the proton such a proton will be deshielded. 39
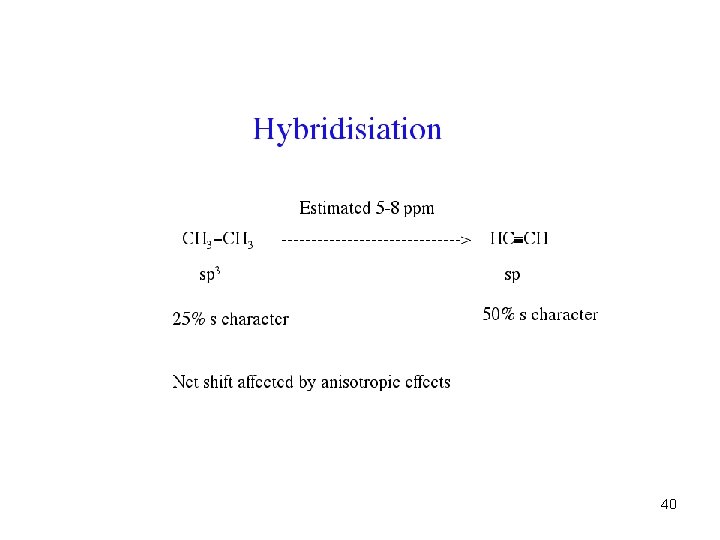
40
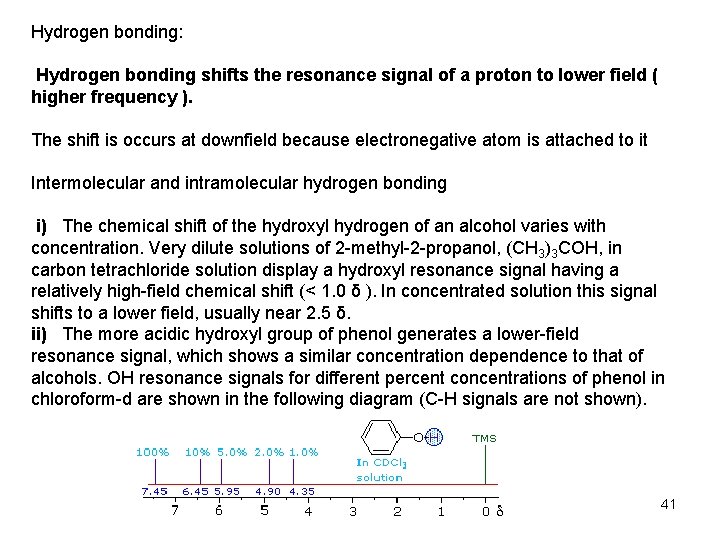
Hydrogen bonding: Hydrogen bonding shifts the resonance signal of a proton to lower field ( higher frequency ). The shift is occurs at downfield because electronegative atom is attached to it Intermolecular and intramolecular hydrogen bonding i) The chemical shift of the hydroxyl hydrogen of an alcohol varies with concentration. Very dilute solutions of 2 -methyl-2 -propanol, (CH 3)3 COH, in carbon tetrachloride solution display a hydroxyl resonance signal having a relatively high-field chemical shift (< 1. 0 δ ). In concentrated solution this signal shifts to a lower field, usually near 2. 5 δ. ii) The more acidic hydroxyl group of phenol generates a lower-field resonance signal, which shows a similar concentration dependence to that of alcohols. OH resonance signals for different percent concentrations of phenol in chloroform-d are shown in the following diagram (C-H signals are not shown). 41
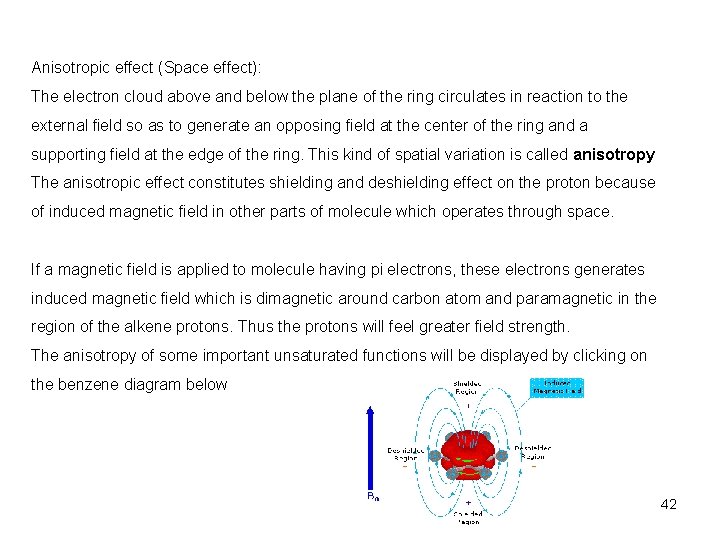
Anisotropic effect (Space effect): The electron cloud above and below the plane of the ring circulates in reaction to the external field so as to generate an opposing field at the center of the ring and a supporting field at the edge of the ring. This kind of spatial variation is called anisotropy The anisotropic effect constitutes shielding and deshielding effect on the proton because of induced magnetic field in other parts of molecule which operates through space. If a magnetic field is applied to molecule having pi electrons, these electrons generates induced magnetic field which is dimagnetic around carbon atom and paramagnetic in the region of the alkene protons. Thus the protons will feel greater field strength. The anisotropy of some important unsaturated functions will be displayed by clicking on the benzene diagram below 42
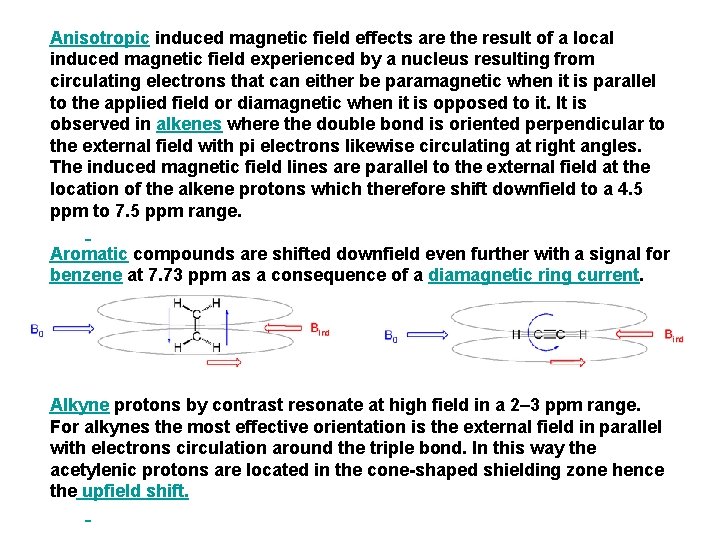
Anisotropic induced magnetic field effects are the result of a local induced magnetic field experienced by a nucleus resulting from circulating electrons that can either be paramagnetic when it is parallel to the applied field or diamagnetic when it is opposed to it. It is observed in alkenes where the double bond is oriented perpendicular to the external field with pi electrons likewise circulating at right angles. The induced magnetic field lines are parallel to the external field at the location of the alkene protons which therefore shift downfield to a 4. 5 ppm to 7. 5 ppm range. Aromatic compounds are shifted downfield even further with a signal for benzene at 7. 73 ppm as a consequence of a diamagnetic ring current. Alkyne protons by contrast resonate at high field in a 2– 3 ppm range. For alkynes the most effective orientation is the external field in parallel with electrons circulation around the triple bond. In this way the acetylenic protons are located in the cone-shaped shielding zone hence the upfield shift.
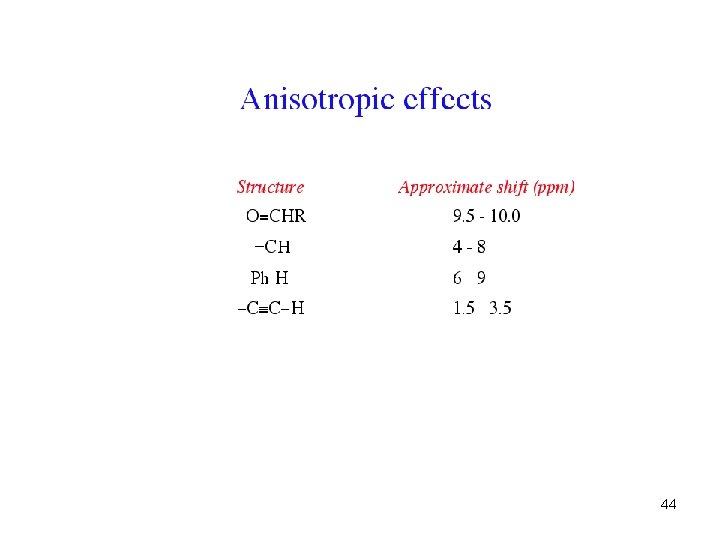
44
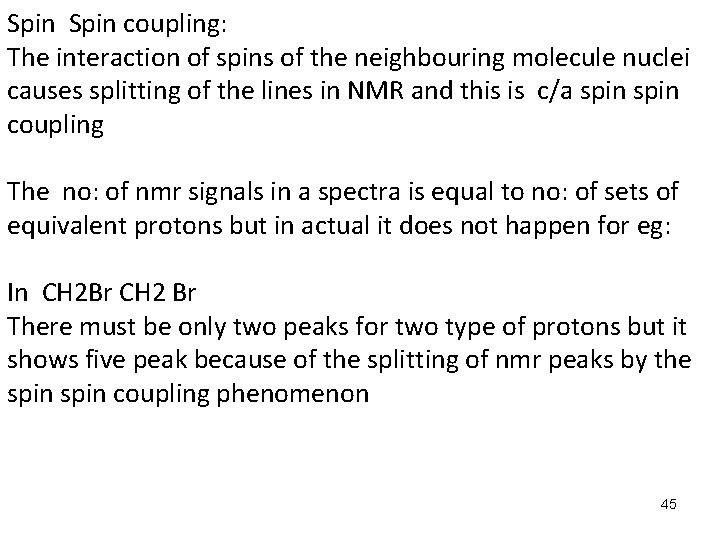
Spin coupling: The interaction of spins of the neighbouring molecule nuclei causes splitting of the lines in NMR and this is c/a spin coupling The no: of nmr signals in a spectra is equal to no: of sets of equivalent protons but in actual it does not happen for eg: In CH 2 Br CH 2 Br There must be only two peaks for two type of protons but it shows five peak because of the splitting of nmr peaks by the spin coupling phenomenon 45
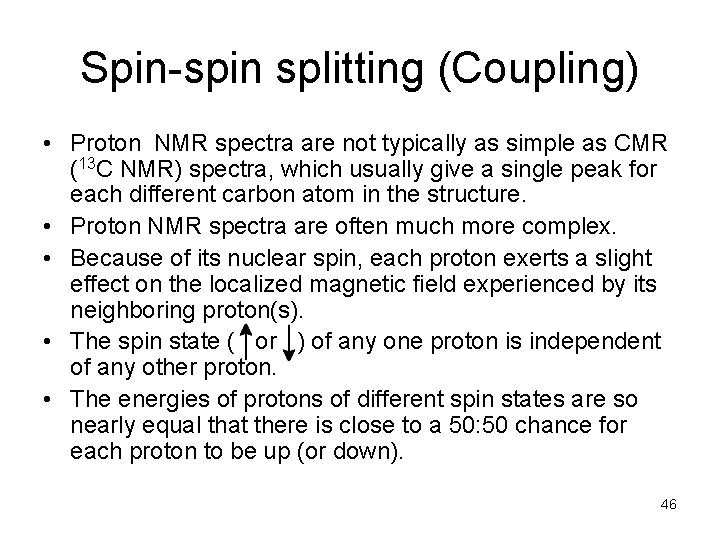
Spin-spin splitting (Coupling) • Proton NMR spectra are not typically as simple as CMR (13 C NMR) spectra, which usually give a single peak for each different carbon atom in the structure. • Proton NMR spectra are often much more complex. • Because of its nuclear spin, each proton exerts a slight effect on the localized magnetic field experienced by its neighboring proton(s). • The spin state ( or ) of any one proton is independent of any other proton. • The energies of protons of different spin states are so nearly equal that there is close to a 50: 50 chance for each proton to be up (or down). 46
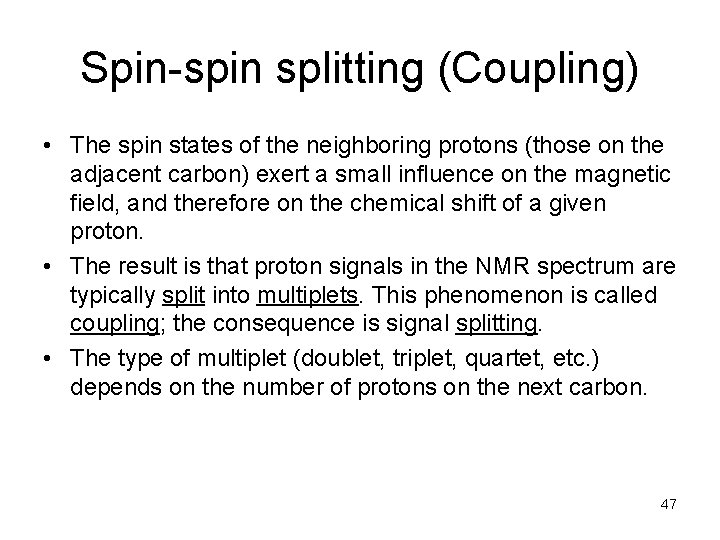
Spin-spin splitting (Coupling) • The spin states of the neighboring protons (those on the adjacent carbon) exert a small influence on the magnetic field, and therefore on the chemical shift of a given proton. • The result is that proton signals in the NMR spectrum are typically split into multiplets. This phenomenon is called coupling; the consequence is signal splitting. • The type of multiplet (doublet, triplet, quartet, etc. ) depends on the number of protons on the next carbon. 47
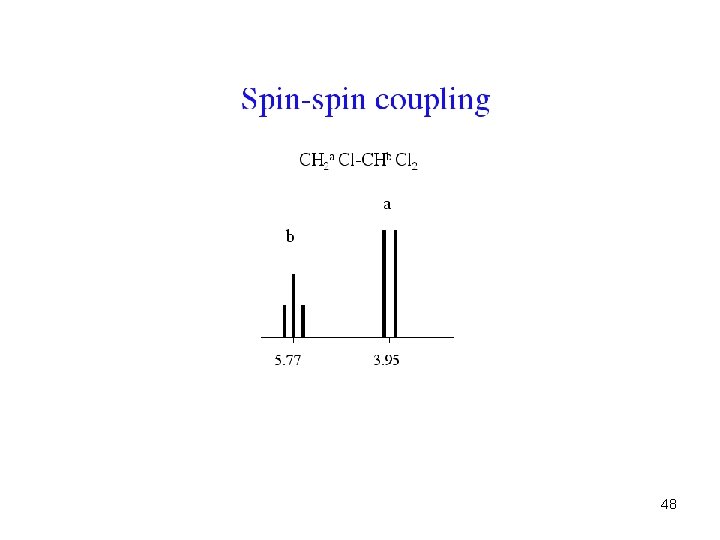
48
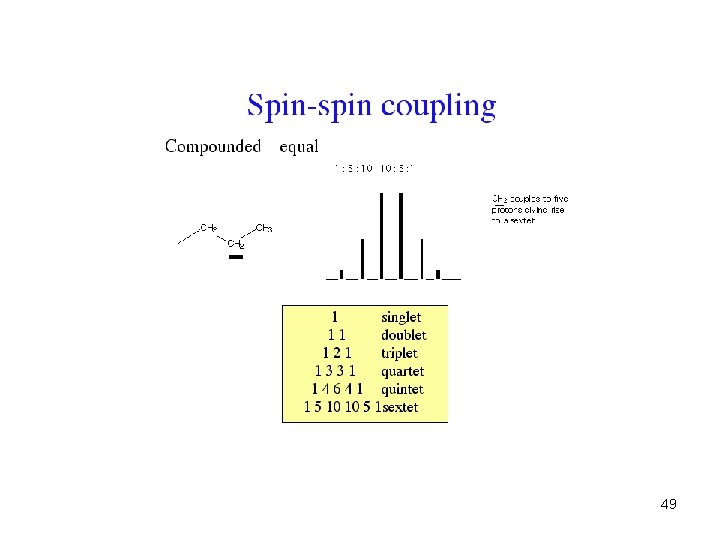
49
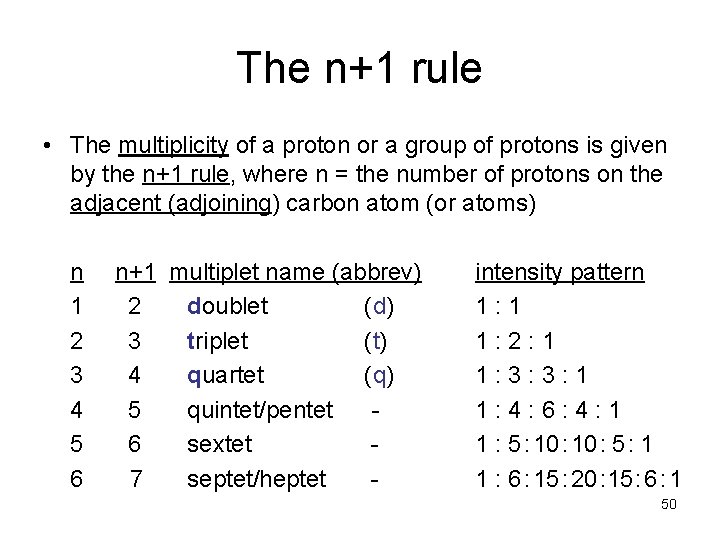
The n+1 rule • The multiplicity of a proton or a group of protons is given by the n+1 rule, where n = the number of protons on the adjacent (adjoining) carbon atom (or atoms) n 1 2 3 4 5 6 n+1 multiplet name (abbrev) 2 doublet (d) 3 triplet (t) 4 quartet (q) 5 quintet/pentet 6 sextet 7 septet/heptet - intensity pattern 1: 1 1: 2: 1 1: 3: 3: 1 1: 4: 6: 4: 1 1 : 5 : 10 : 5 : 1 1 : 6 : 15 : 20 : 15 : 6 : 1 50
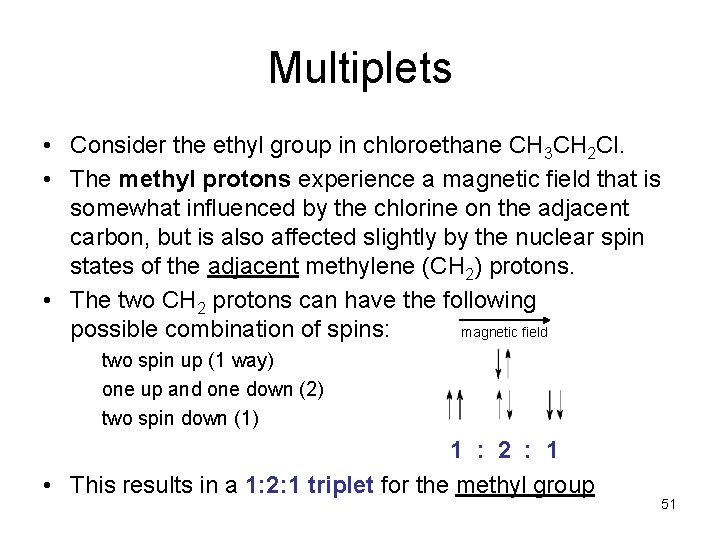
Multiplets • Consider the ethyl group in chloroethane CH 3 CH 2 Cl. • The methyl protons experience a magnetic field that is somewhat influenced by the chlorine on the adjacent carbon, but is also affected slightly by the nuclear spin states of the adjacent methylene (CH 2) protons. • The two CH 2 protons can have the following possible combination of spins: magnetic field two spin up (1 way) one up and one down (2) two spin down (1) 1 : 2 : 1. • This results in a 1: 2: 1 triplet for the methyl group 51
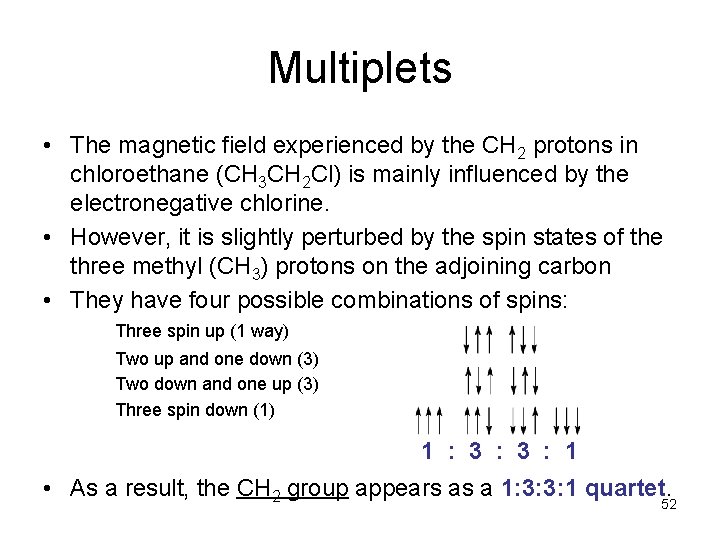
Multiplets • The magnetic field experienced by the CH 2 protons in chloroethane (CH 3 CH 2 Cl) is mainly influenced by the electronegative chlorine. • However, it is slightly perturbed by the spin states of the three methyl (CH 3) protons on the adjoining carbon • They have four possible combinations of spins: Three spin up (1 way) Two up and one down (3) Two down and one up (3) Three spin down (1) 1 : 3 : 1 • As a result, the CH 2 group appears as a 1: 3: 3: 1 quartet. 52
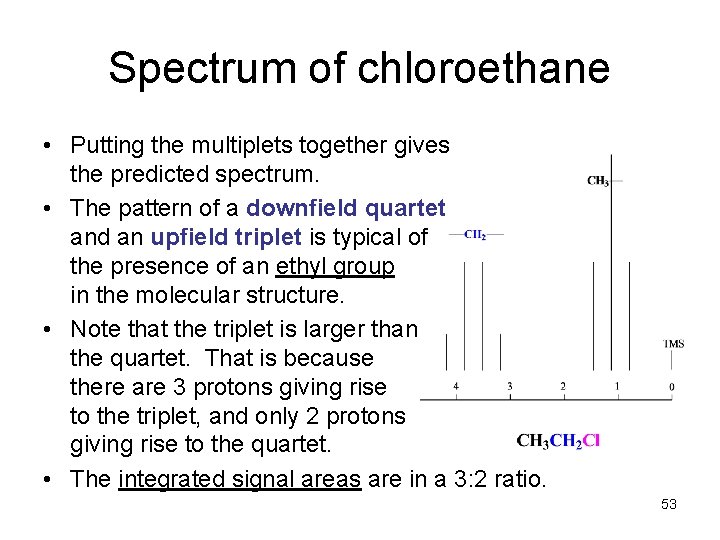
Spectrum of chloroethane • Putting the multiplets together gives the predicted spectrum. • The pattern of a downfield quartet and an upfield triplet is typical of the presence of an ethyl group in the molecular structure. • Note that the triplet is larger than the quartet. That is because there are 3 protons giving rise to the triplet, and only 2 protons giving rise to the quartet. • The integrated signal areas are in a 3: 2 ratio. 53
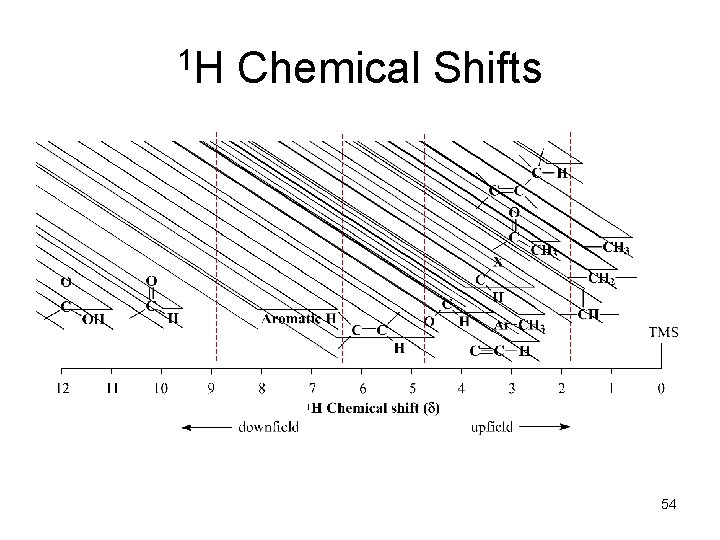
1 H Chemical Shifts 54
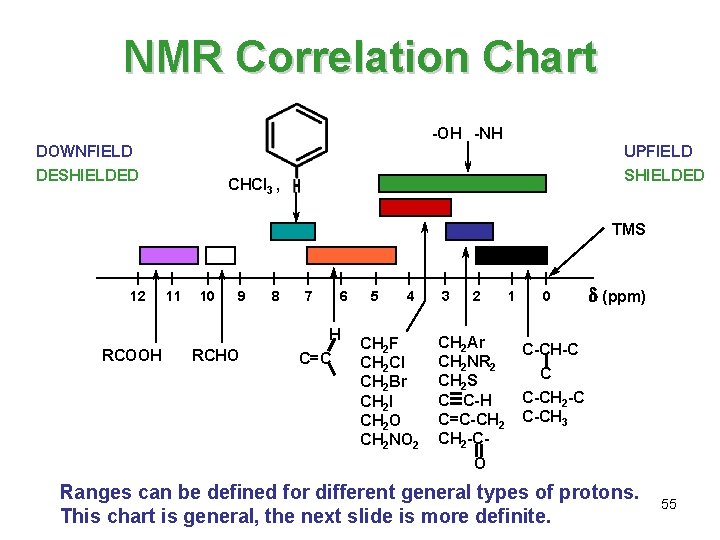
NMR Correlation Chart -OH -NH DOWNFIELD DESHIELDED UPFIELD SHIELDED CHCl 3 , TMS 12 RCOOH 11 10 9 RCHO 8 7 6 H C=C 5 4 CH 2 F CH 2 Cl CH 2 Br CH 2 I CH 2 O CH 2 NO 2 3 2 CH 2 Ar CH 2 NR 2 CH 2 S C C-H C=C-CH 2 -C- 1 0 d (ppm) C-CH-C C C-CH 2 -C C-CH 3 O Ranges can be defined for different general types of protons. This chart is general, the next slide is more definite. 55
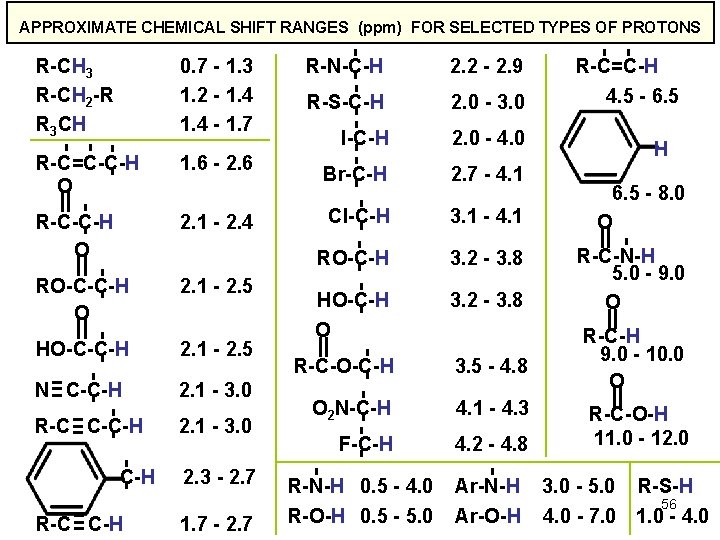
APPROXIMATE CHEMICAL SHIFT RANGES (ppm) FOR SELECTED TYPES OF PROTONS R-CH 3 R-CH 2 -R R 3 CH 0. 7 - 1. 3 1. 2 - 1. 4 - 1. 7 R-C=C-C-H O 1. 6 - 2. 6 R-C-C-H O 2. 1 - 2. 4 RO-C-C-H O 2. 1 - 2. 5 HO-C-C-H 2. 1 - 2. 5 N C-C-H 2. 1 - 3. 0 R-C C-C-H 2. 1 - 3. 0 C-H R-C C-H 2. 3 - 2. 7 1. 7 - 2. 7 R-N-C-H 2. 2 - 2. 9 R-S-C-H 2. 0 - 3. 0 I-C-H 2. 0 - 4. 0 Br-C-H 2. 7 - 4. 1 Cl-C-H 3. 1 - 4. 1 RO-C-H 3. 2 - 3. 8 HO-C-H O 3. 2 - 3. 8 R-C-O-C-H 3. 5 - 4. 8 O 2 N-C-H 4. 1 - 4. 3 F-C-H 4. 2 - 4. 8 R-N-H 0. 5 - 4. 0 R-O-H 0. 5 - 5. 0 Ar-N-H Ar-O-H R-C=C-H 4. 5 - 6. 5 H 6. 5 - 8. 0 O R-C-N-H 5. 0 - 9. 0 O R-C-H 9. 0 - 10. 0 O R-C-O-H 11. 0 - 12. 0 3. 0 - 5. 0 R-S-H 56 4. 0 - 7. 0 1. 0 - 4. 0
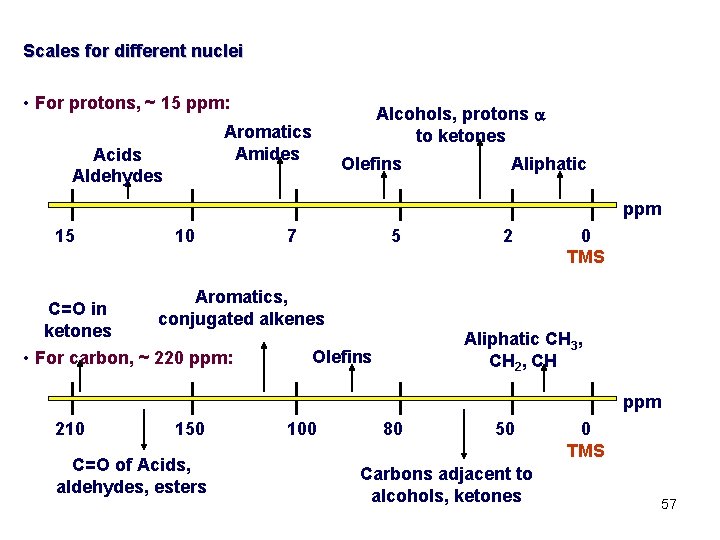
Scales for different nuclei • For protons, ~ 15 ppm: Aromatics Amides Acids Aldehydes Alcohols, protons a to ketones Olefins Aliphatic ppm 15 C=O in ketones 10 7 5 2 0 TMS Aromatics, conjugated alkenes • For carbon, ~ 220 ppm: Aliphatic CH 3, CH 2, CH Olefins ppm 210 150 C=O of Acids, aldehydes, esters 100 80 50 Carbons adjacent to alcohols, ketones 0 TMS 57
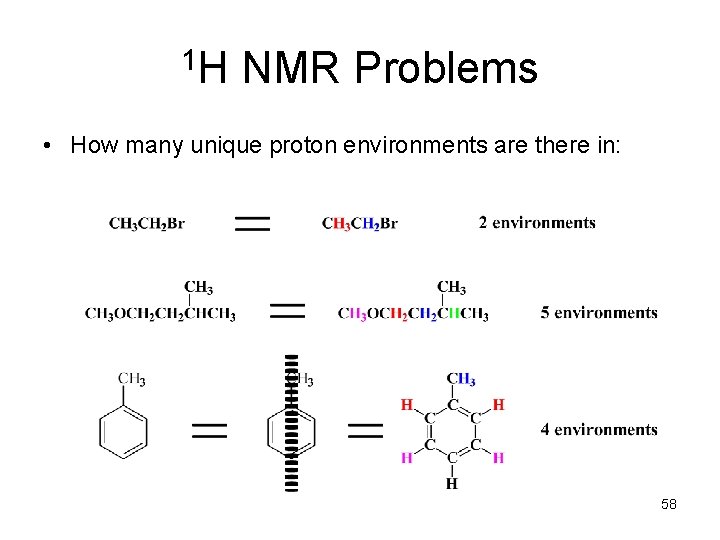
1 H NMR Problems • How many unique proton environments are there in: 58
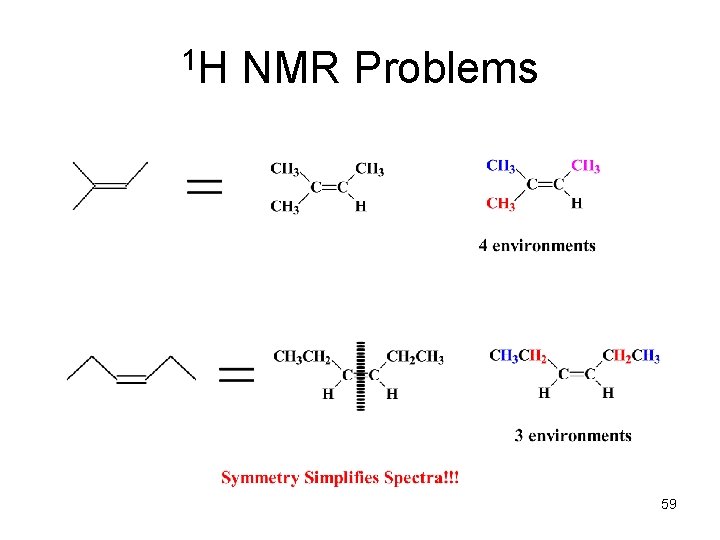
1 H NMR Problems 59
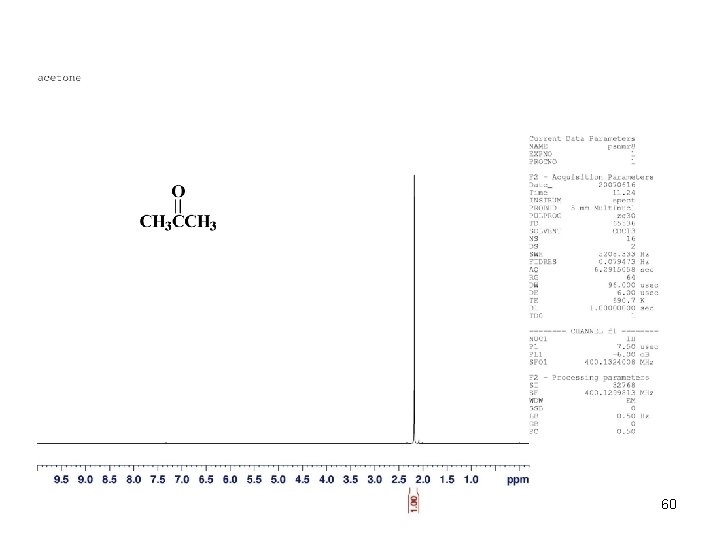
60
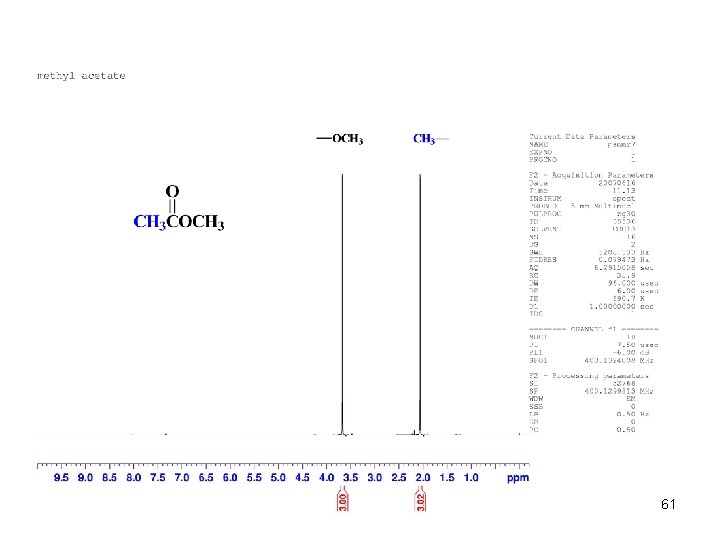
61
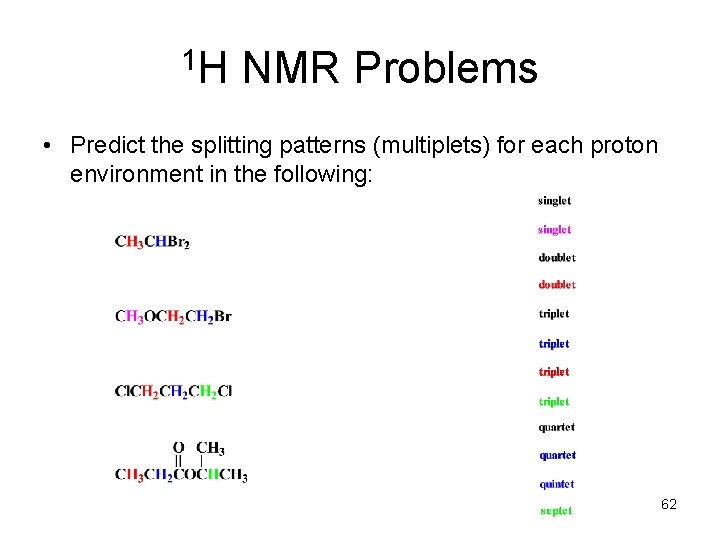
1 H NMR Problems • Predict the splitting patterns (multiplets) for each proton environment in the following: 62
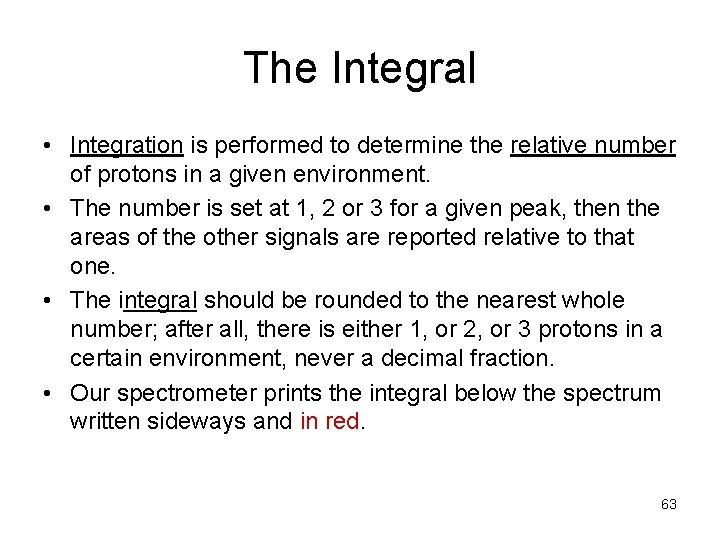
The Integral • Integration is performed to determine the relative number of protons in a given environment. • The number is set at 1, 2 or 3 for a given peak, then the areas of the other signals are reported relative to that one. • The integral should be rounded to the nearest whole number; after all, there is either 1, or 2, or 3 protons in a certain environment, never a decimal fraction. • Our spectrometer prints the integral below the spectrum written sideways and in red. 63
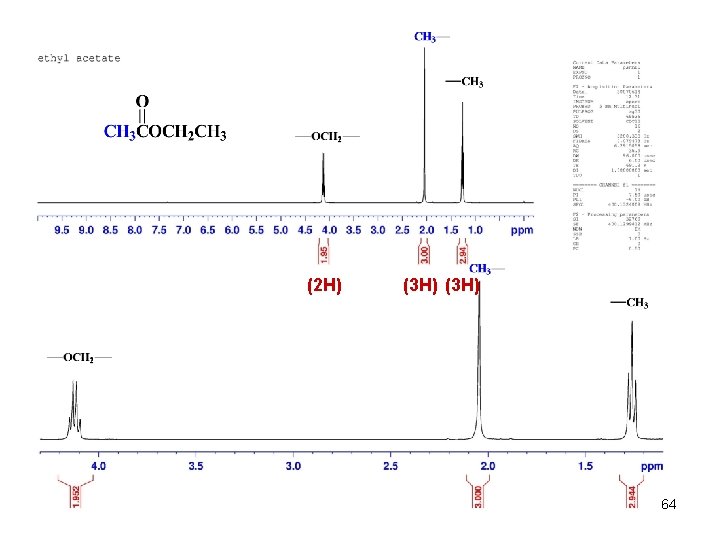
(2 H) (3 H) 64
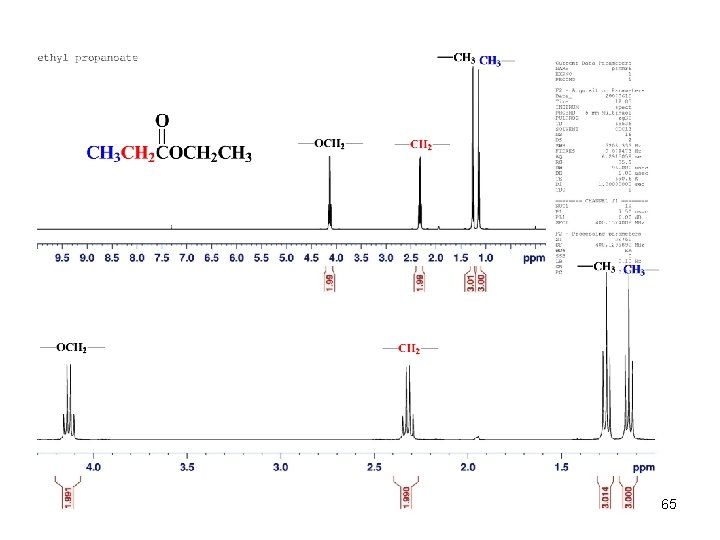
65
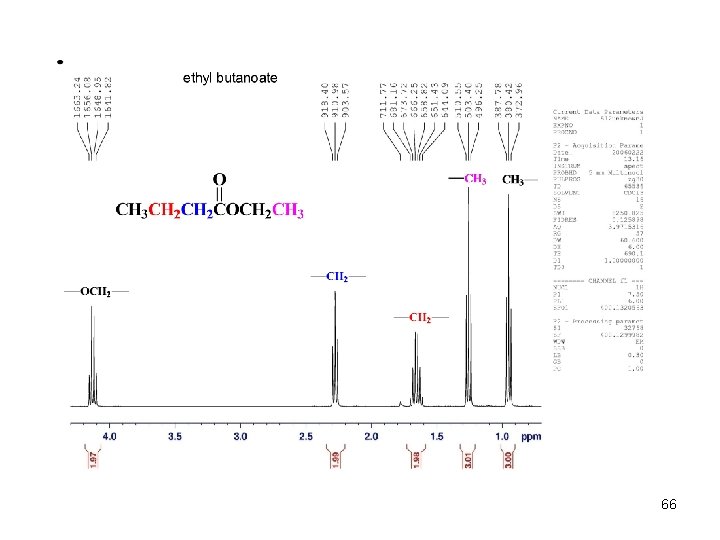
C 6 H 12 O 2 66
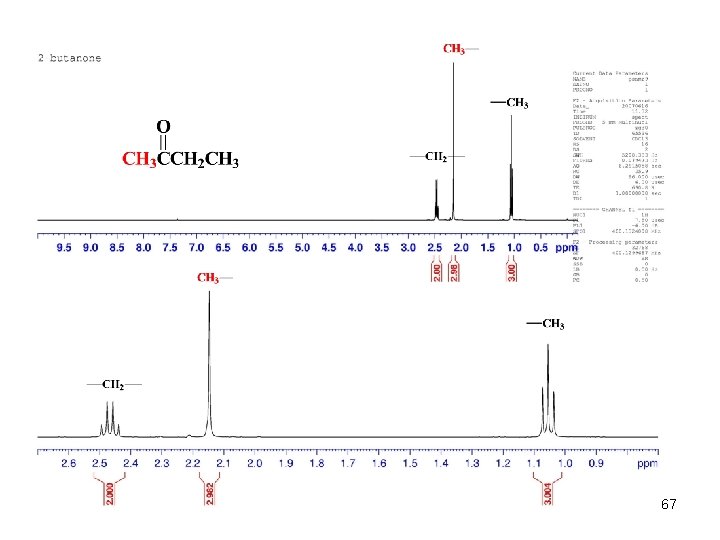
67
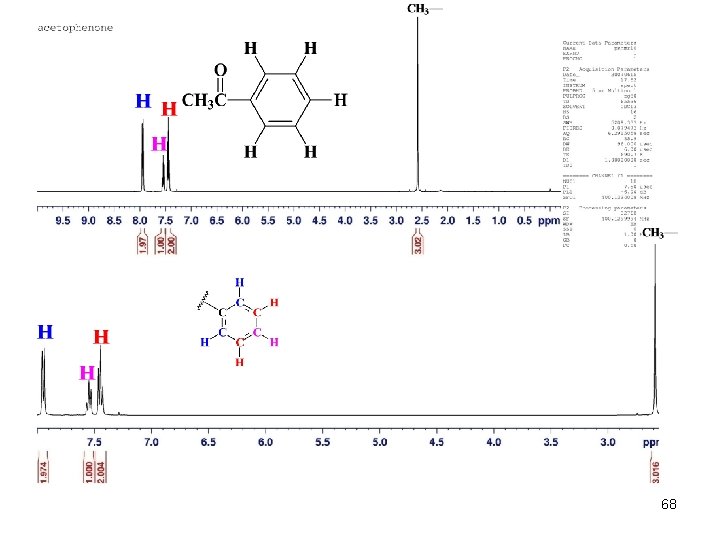
68
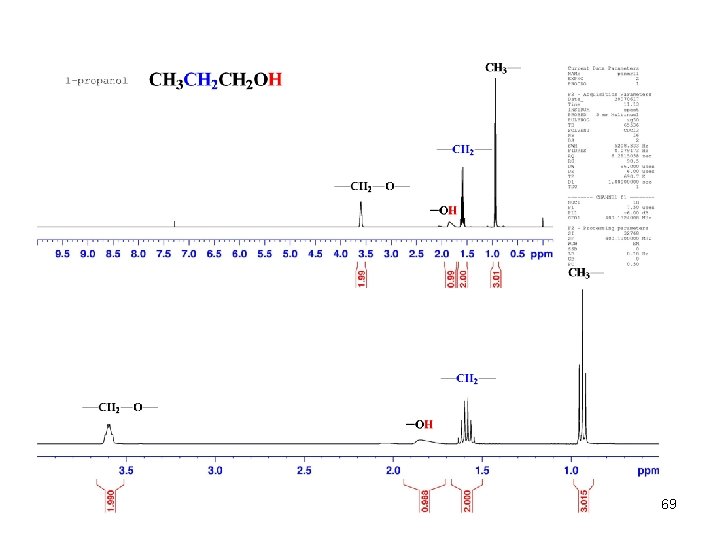
69
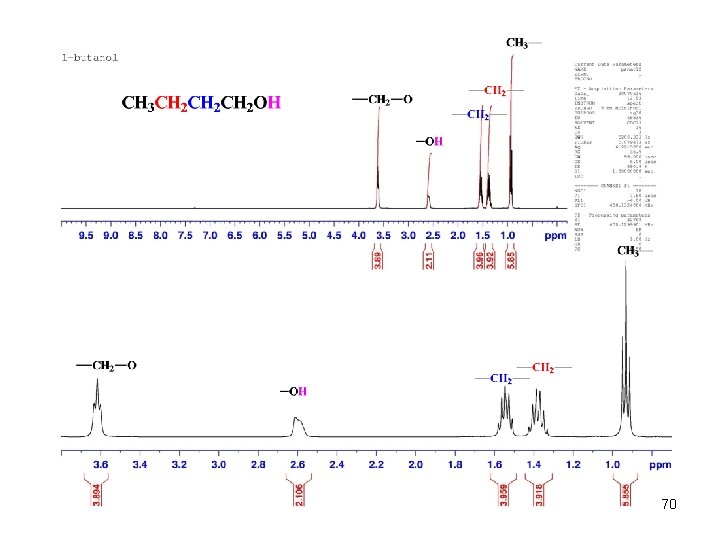
70
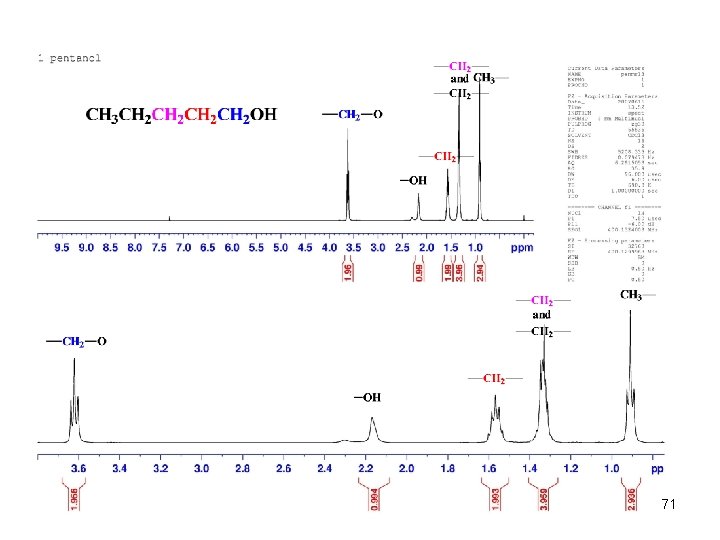
71
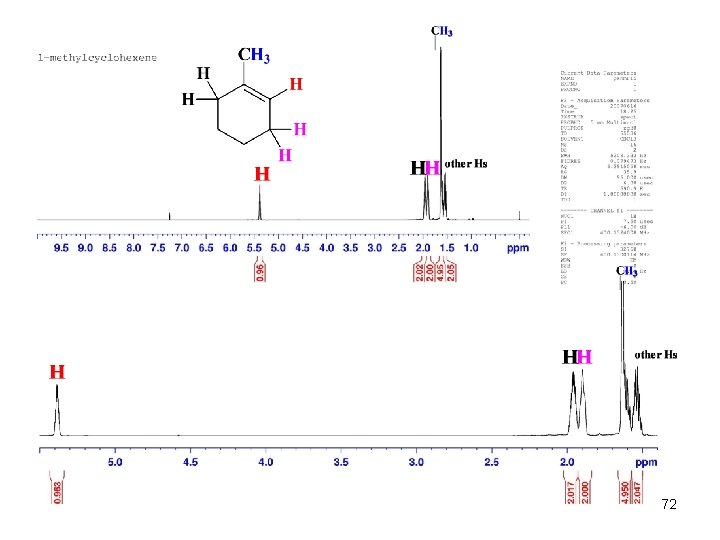
72
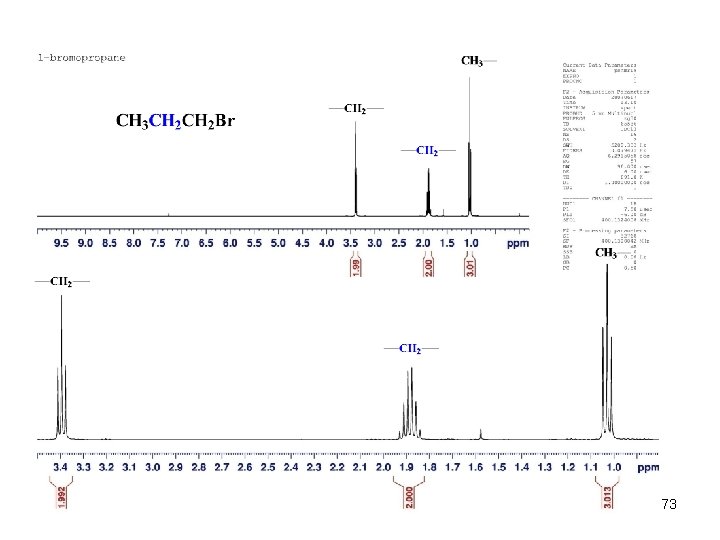
73
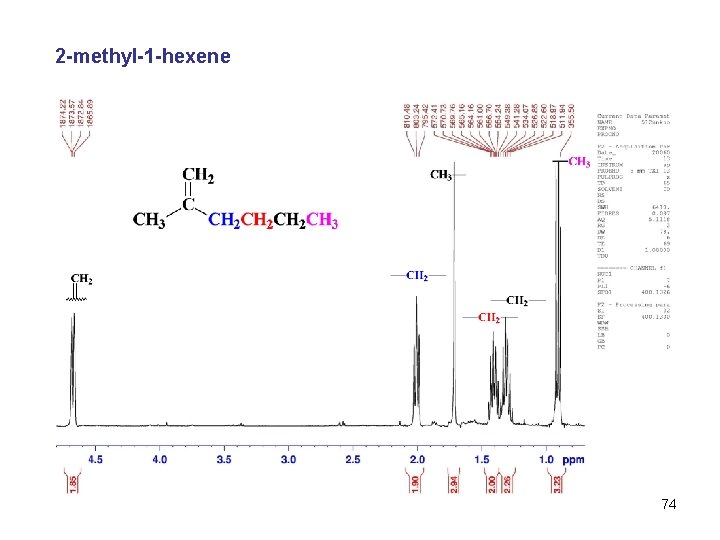
2 -methyl-1 -hexene 74
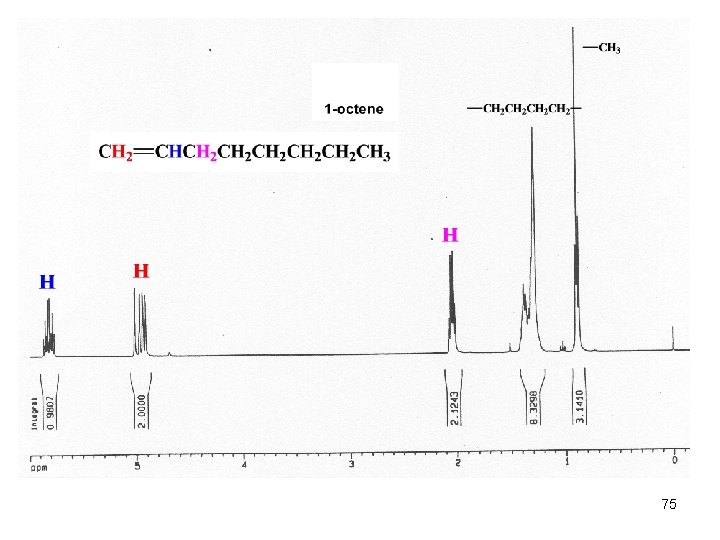
C 8 H 16 75
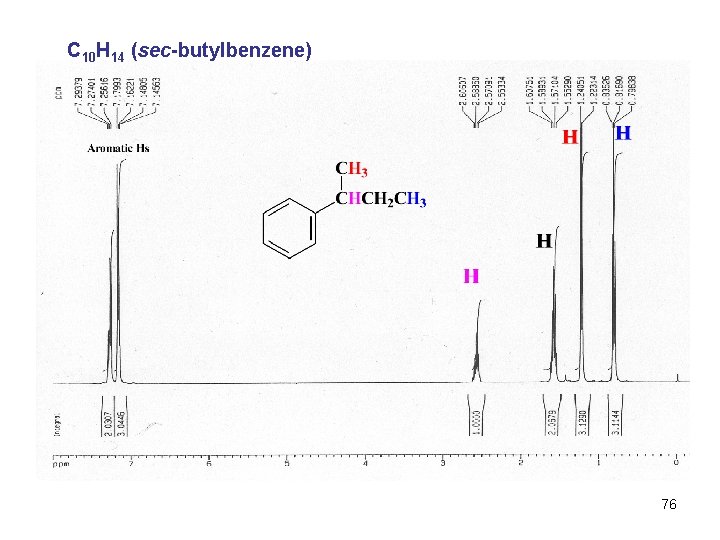
C 10 H 14 (sec-butylbenzene) 76
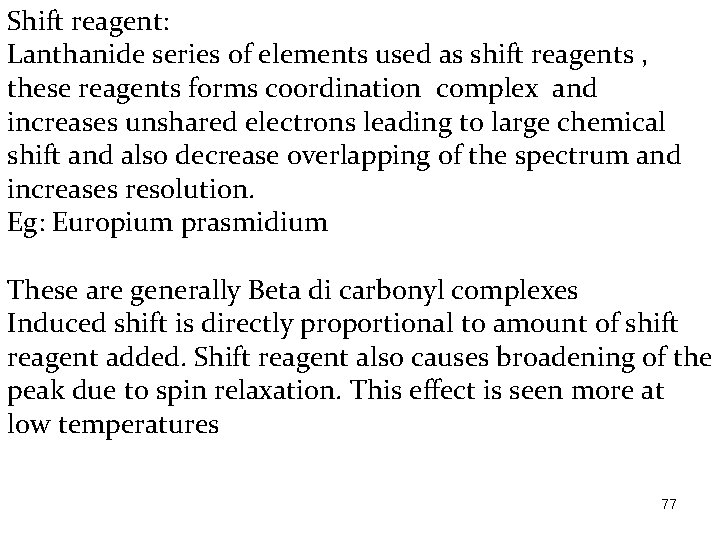
Shift reagent: Lanthanide series of elements used as shift reagents , these reagents forms coordination complex and increases unshared electrons leading to large chemical shift and also decrease overlapping of the spectrum and increases resolution. Eg: Europium prasmidium These are generally Beta di carbonyl complexes Induced shift is directly proportional to amount of shift reagent added. Shift reagent also causes broadening of the peak due to spin relaxation. This effect is seen more at low temperatures 77
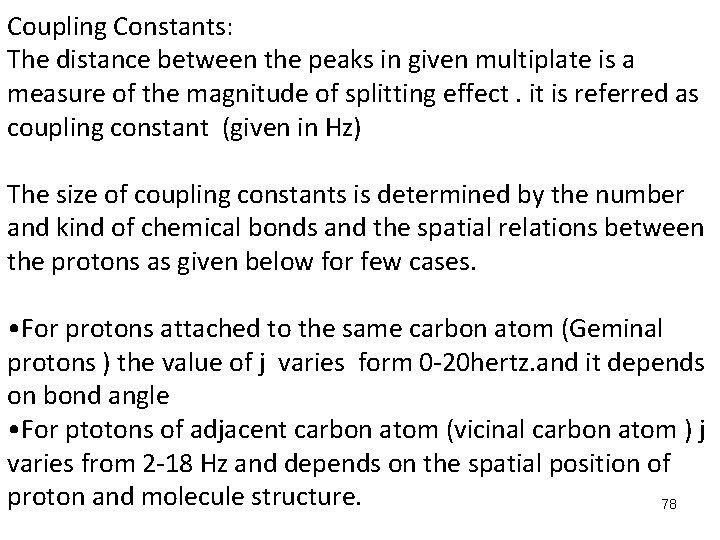
Coupling Constants: The distance between the peaks in given multiplate is a measure of the magnitude of splitting effect. it is referred as coupling constant (given in Hz) The size of coupling constants is determined by the number and kind of chemical bonds and the spatial relations between the protons as given below for few cases. • For protons attached to the same carbon atom (Geminal protons ) the value of j varies form 0 -20 hertz. and it depends on bond angle • For ptotons of adjacent carbon atom (vicinal carbon atom ) j varies from 2 -18 Hz and depends on the spatial position of proton and molecule structure. 78
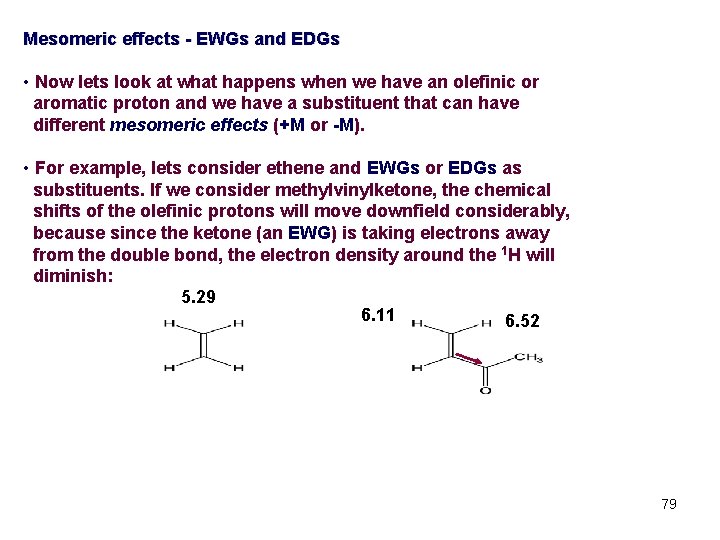
Mesomeric effects - EWGs and EDGs • Now lets look at what happens when we have an olefinic or aromatic proton and we have a substituent that can have different mesomeric effects (+M or -M). • For example, lets consider ethene and EWGs or EDGs as substituents. If we consider methylvinylketone, the chemical shifts of the olefinic protons will move downfield considerably, because since the ketone (an EWG) is taking electrons away from the double bond, the electron density around the 1 H will diminish: 5. 29 6. 11 6. 52 79
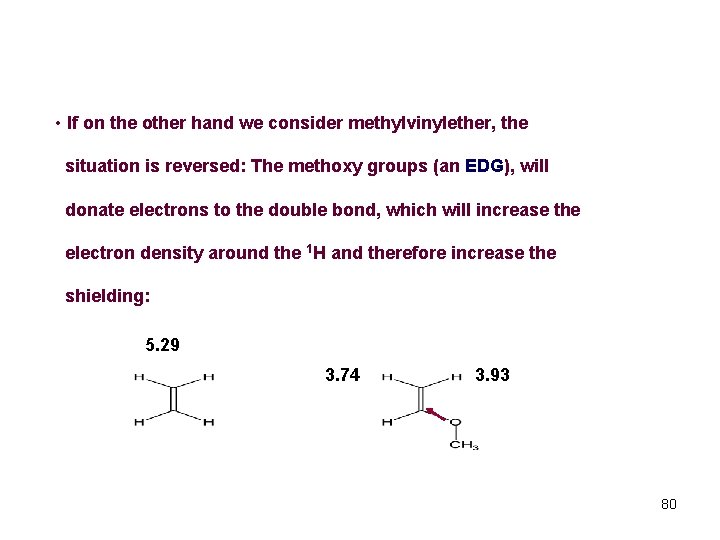
• If on the other hand we consider methylvinylether, the situation is reversed: The methoxy groups (an EDG), will donate electrons to the double bond, which will increase the electron density around the 1 H and therefore increase the shielding: 5. 29 3. 74 3. 93 80
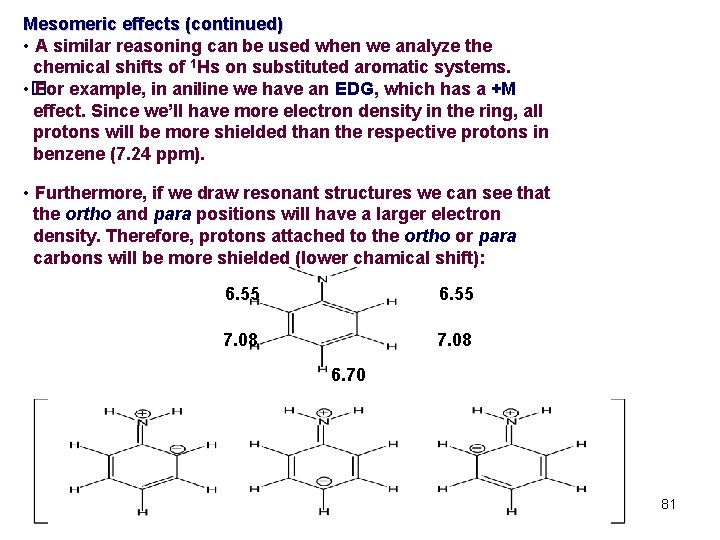
Mesomeric effects (continued) • A similar reasoning can be used when we analyze the chemical shifts of 1 Hs on substituted aromatic systems. • � For example, in aniline we have an EDG, which has a +M effect. Since we’ll have more electron density in the ring, all protons will be more shielded than the respective protons in benzene (7. 24 ppm). • Furthermore, if we draw resonant structures we can see that the ortho and para positions will have a larger electron density. Therefore, protons attached to the ortho or para carbons will be more shielded (lower chamical shift): 6. 55 7. 08 6. 70 81
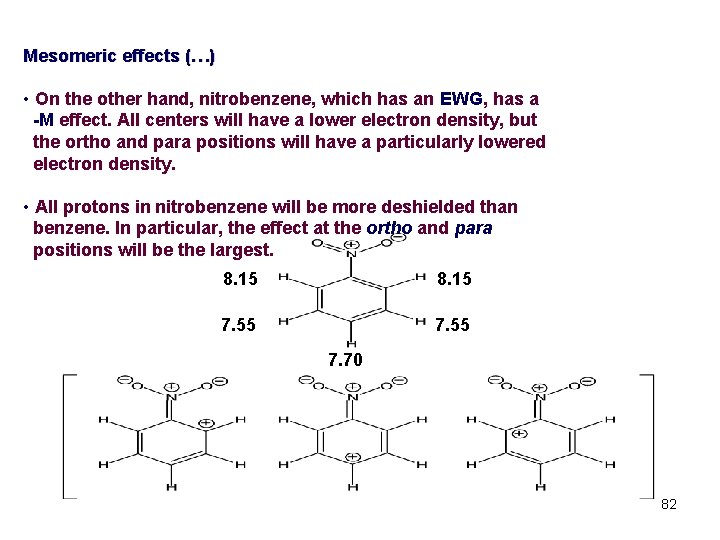
Mesomeric effects (…) • On the other hand, nitrobenzene, which has an EWG, has a -M effect. All centers will have a lower electron density, but the ortho and para positions will have a particularly lowered electron density. • All protons in nitrobenzene will be more deshielded than benzene. In particular, the effect at the ortho and para positions will be the largest. 8. 15 7. 55 7. 70 82
Applications of nuclear magnetic resonance
Spectroscopy
Dept nmr spectroscopy
Advantages of nmr spectroscopy
P-diethylbenzene nmr
Nmr instrument diagram
Geminal and vicinal coupling constants
Magnetic resonance timisoara
Nait tag placement
"magnetic e-resonance therapy"
Evans method magnetic susceptibility
Magnetic moment and magnetic field relation
F=i(lxb)
The coercive force in a ferromagnetic material is
Weber unit
Lesson 15 nuclear quest nuclear reactions
Fisión nuclear vs fision nuclear
Bridge collapse due to resonance
Dullness to percussion
Hcon(ch3)2 lewis structure
Forced vibration and resonance
Molecular rotational resonance
Resonance standing wave
Percussion lungs
Series and parallel circuits
Resonance signal
No arrows
Localized lone pair
Decreased tactile fremitus
Physics resonance
Resonance theory is
Molecular geometry chart
Ocn- resonance
Brand resonance pyramid
Forced vibration and resonance
Formula for formal charge
Chocl lewis structure
Efek resonansi adalah
Electric dipole spin resonance
Resonance structures of co32-
Ncs- lewis structure resonance
Vc
Et 242
Ccl2f2 lewis
Resonance hybrid
Nitric acid formal charge
Demi vie tramadol lp
Resonance hybrid
Glashow resonance
How to calculate formal charge?
Is a triple bond the strongest
Isoquinoline nucleophilic substitution
Tocoma narrows bridge
Coca cola brand resonance pyramid
Brand resonance and the brand value chain
Aromaticity of benzenoid and non benzenoid compounds
Styles of creative advertising
Nike brand resonance
Resonance hybrid
Lewis concept of covalent bond
Ocn- resonance
What is resonance in ac circuit
Auscultation purpose
Delocalization and resonance
2 4-pentanedione resonance structure
What is pipe resonance
Electron spin resonance takes in
Subdimensions of brand building blocks
Isoquinoline resonance
Organic chemistry
Stairs in 3 point perspective
Series resonance circuit
Sound intensity and resonance
Adaptive resonance theory example
Brand architecture pyramid
Bridge collapse due to resonance
Pyridine is heterocyclic compound
Coupled pendulum
Rotational spectral lines
Periklis papadopoulos
Difference between atomic and molecular spectroscopy
Gross selection rules
Selection rules for raman spectroscopy