Heat Transfer Steam Generation in A Furnace P
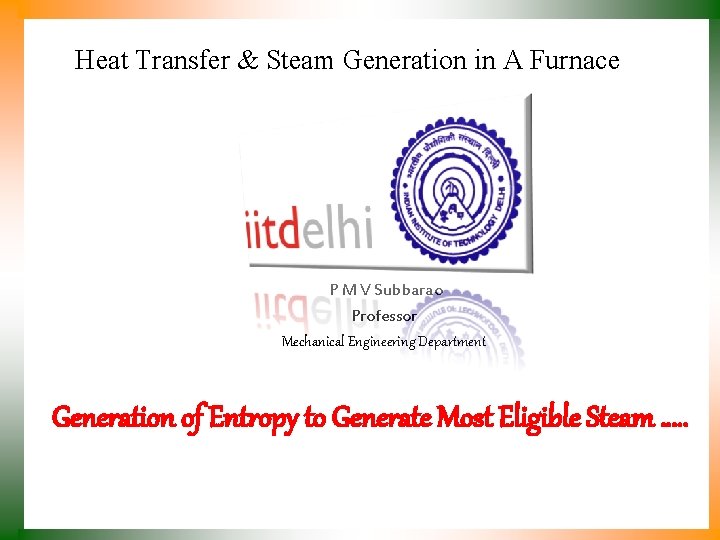
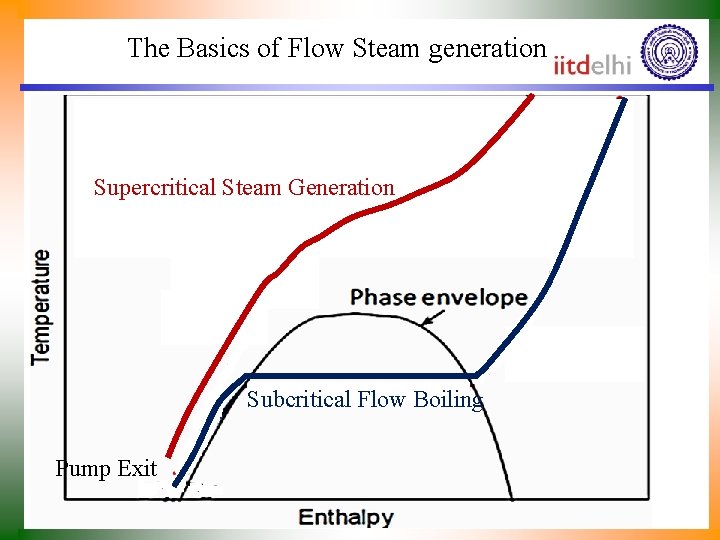
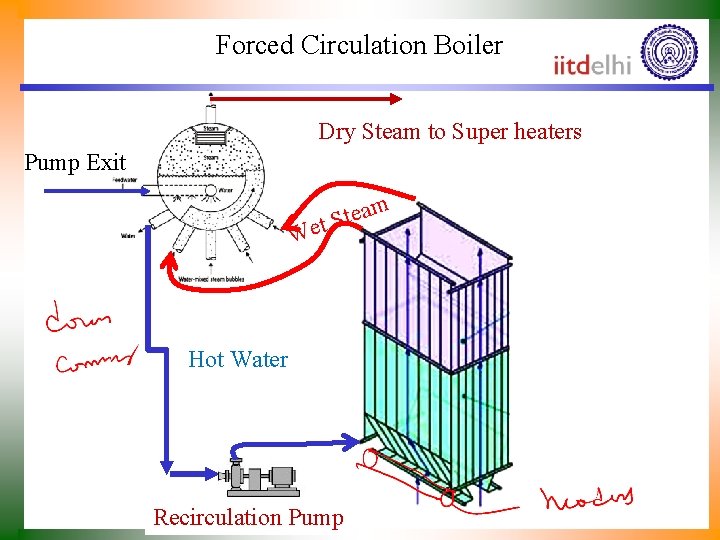
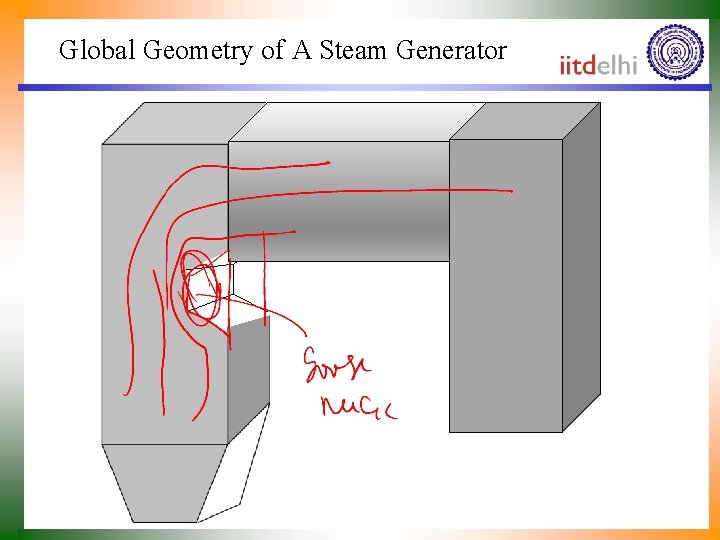
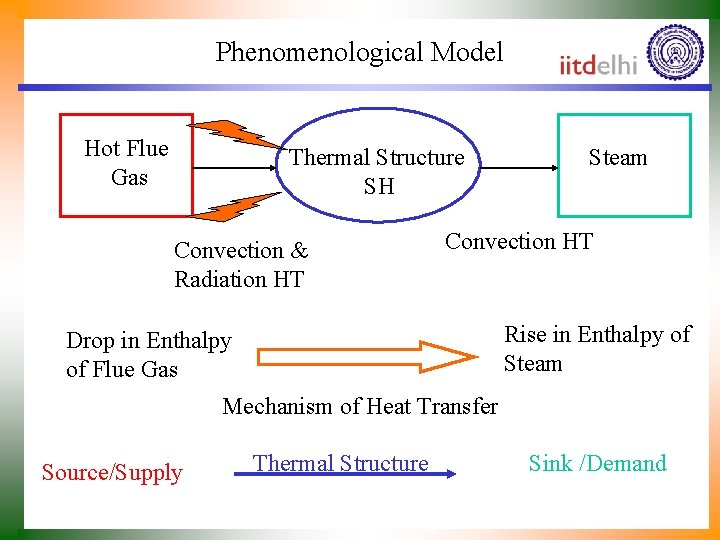
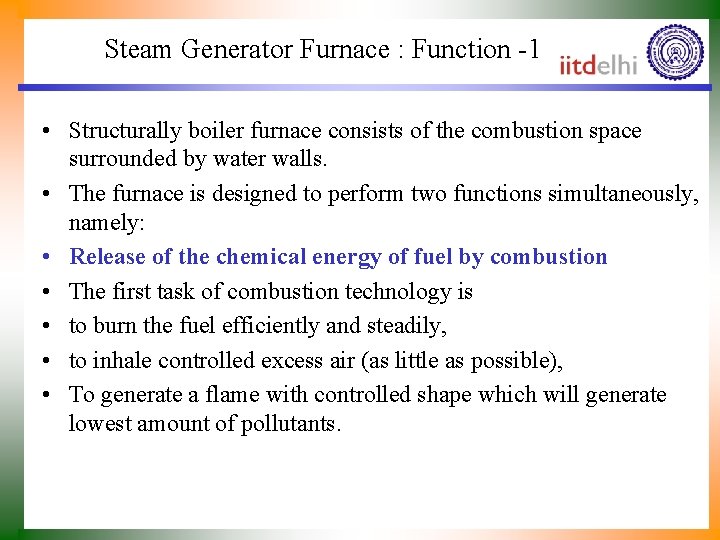
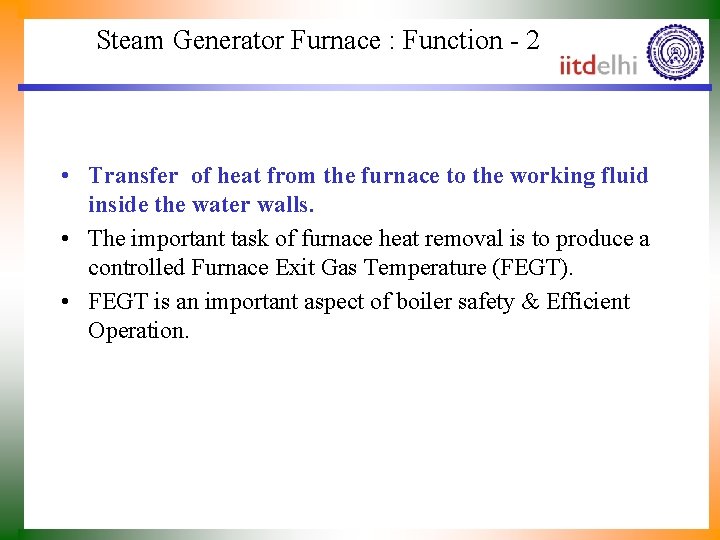
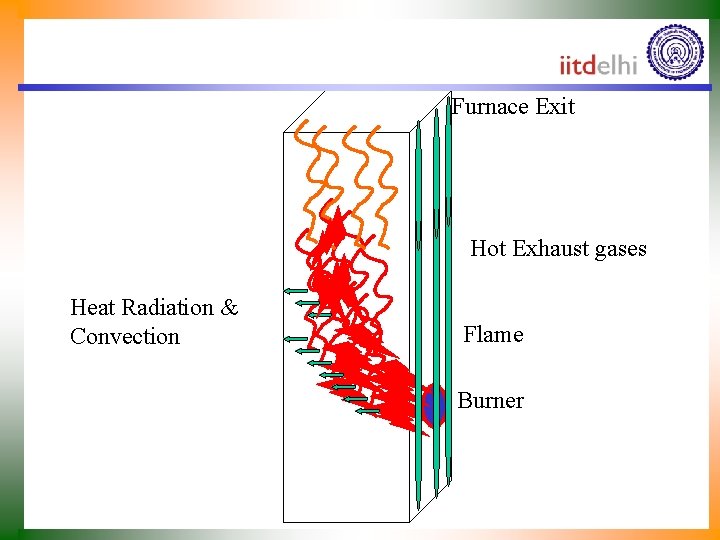
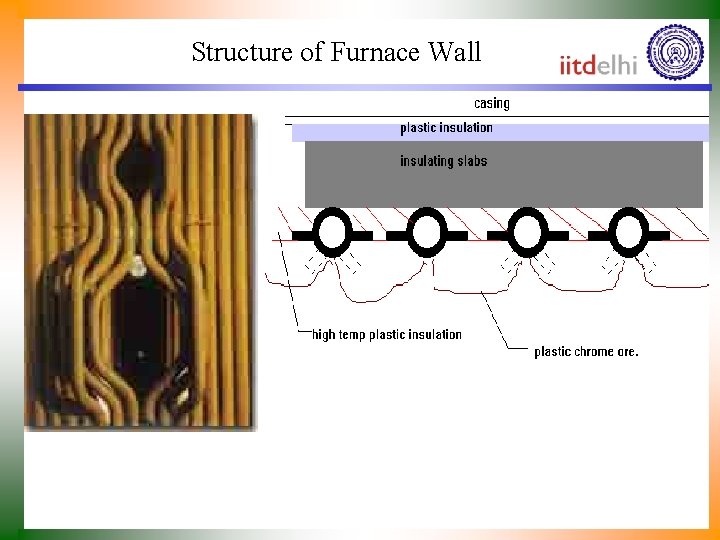
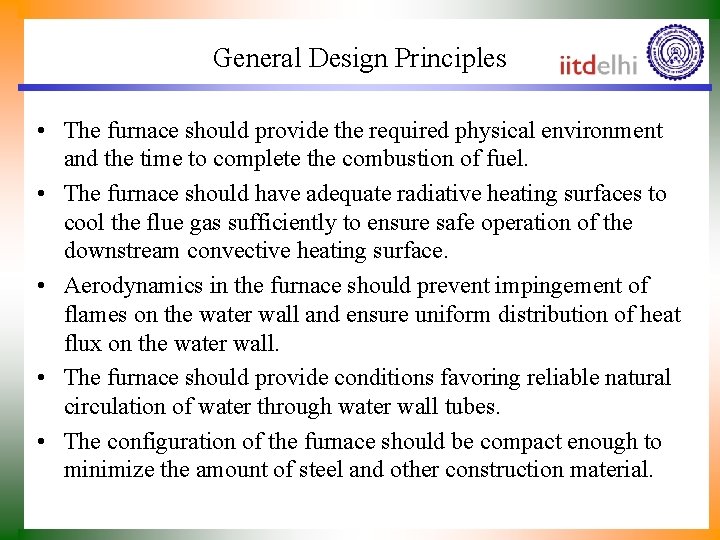
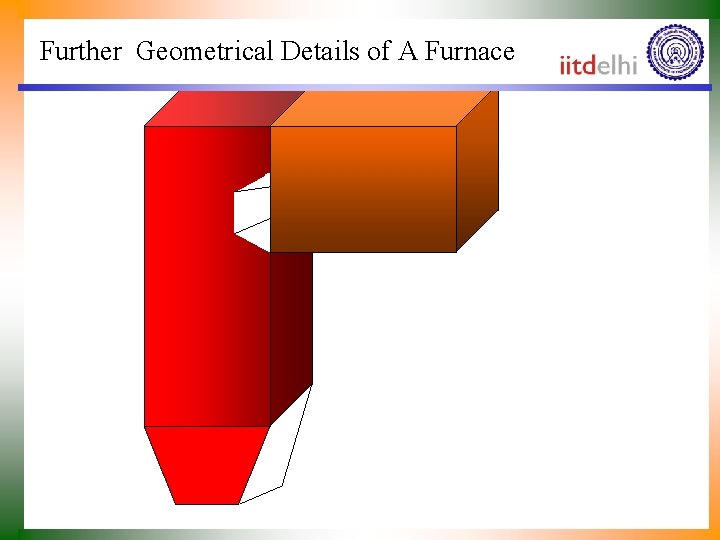
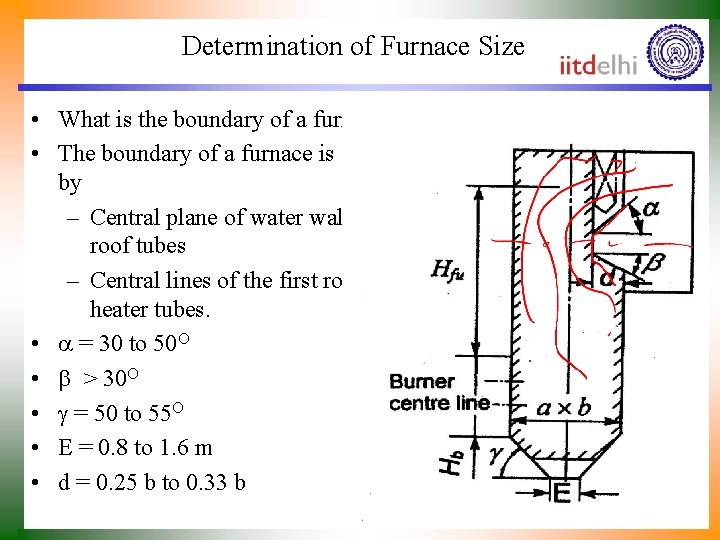
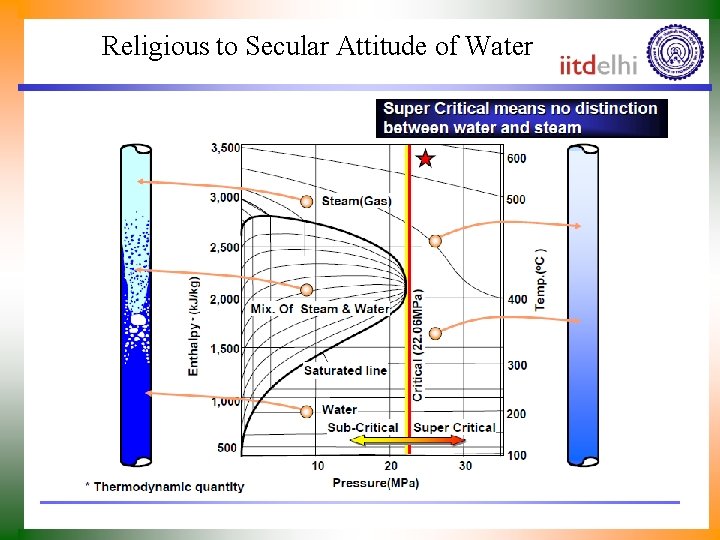
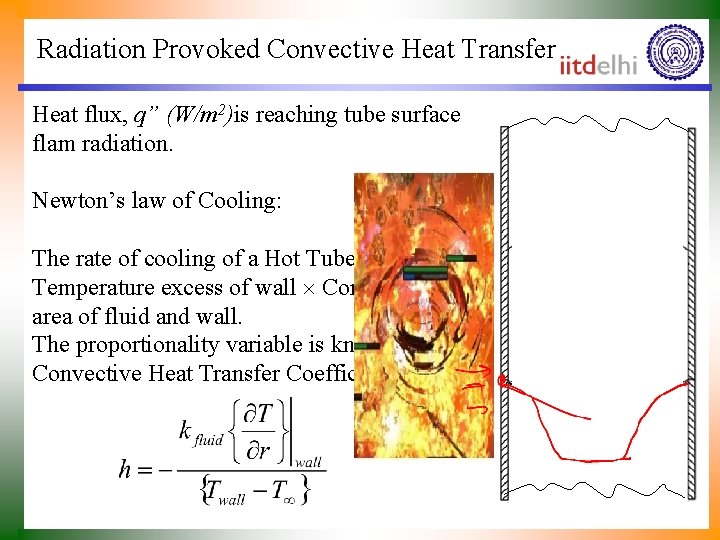
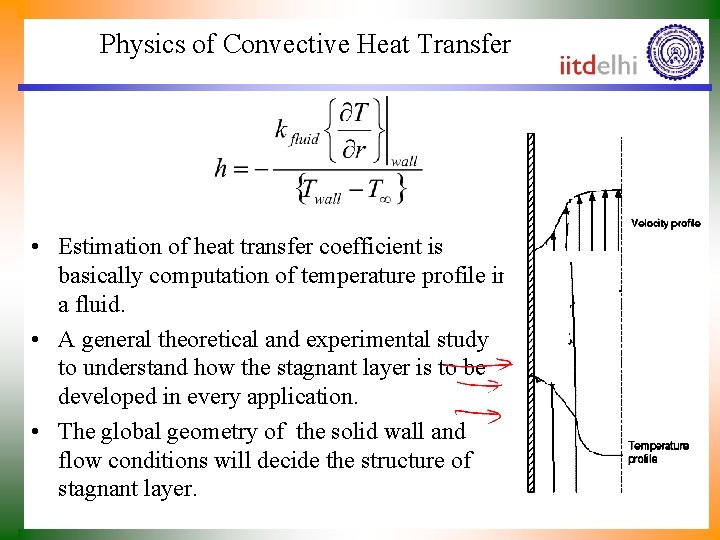
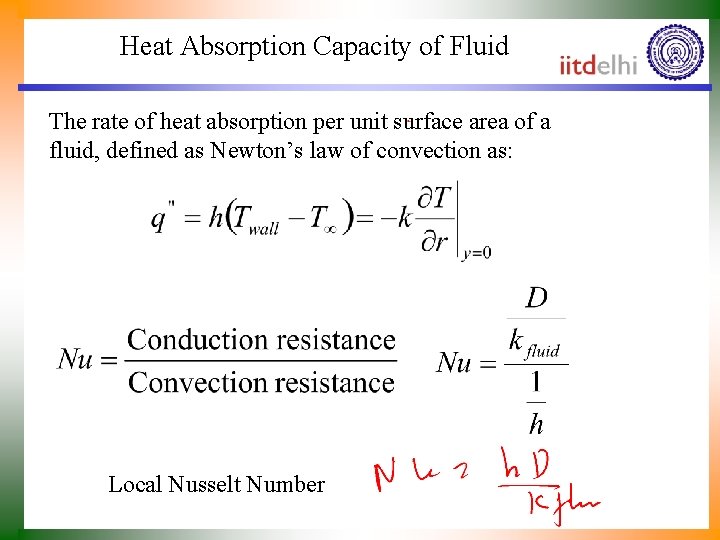
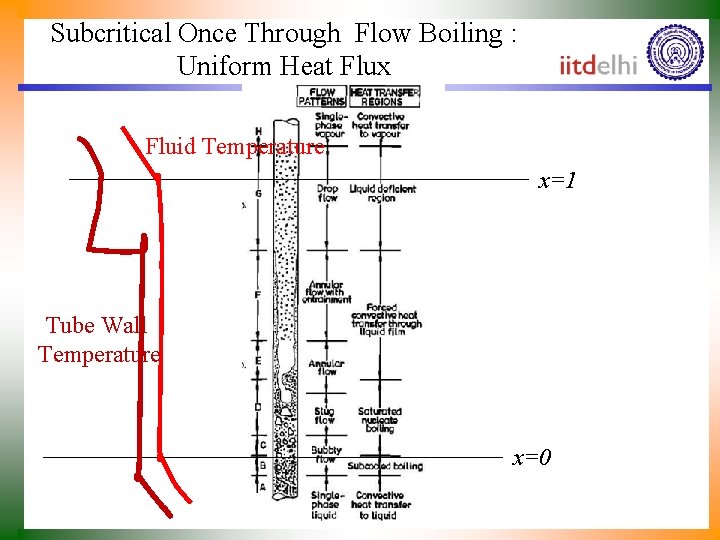
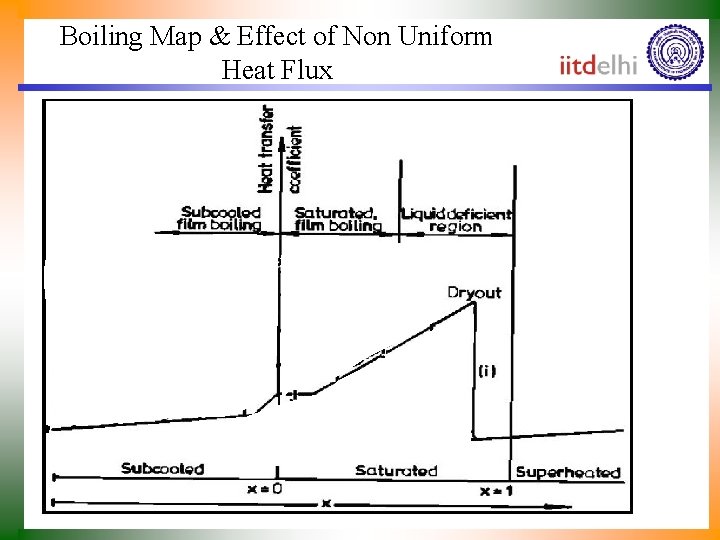
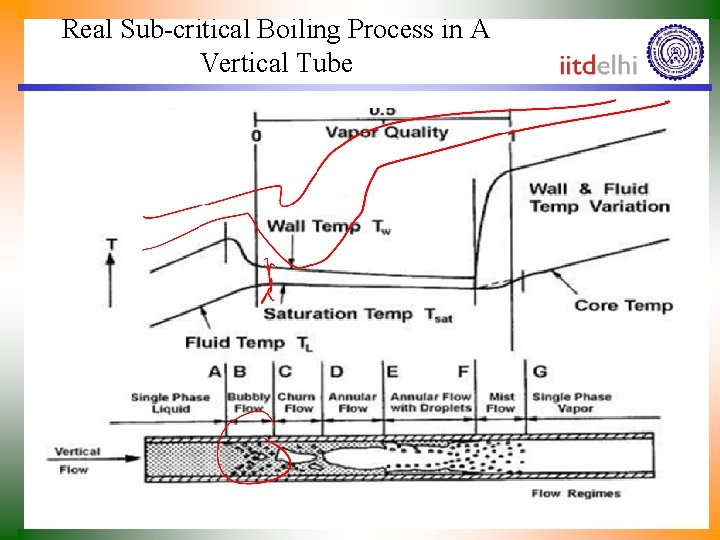
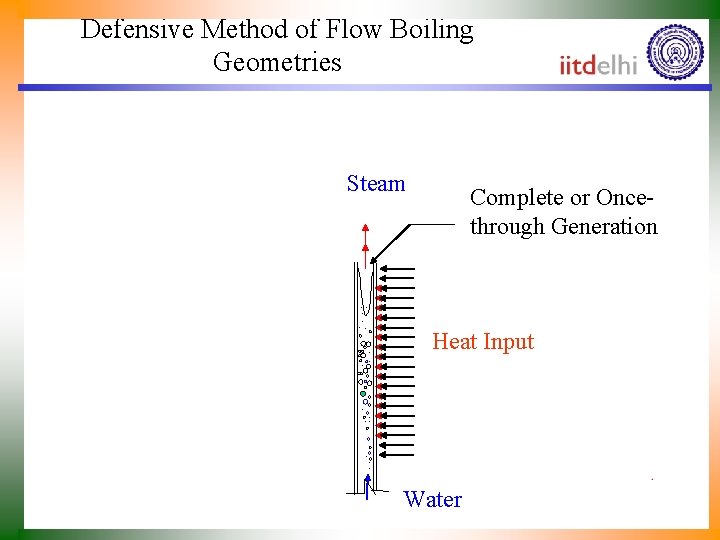
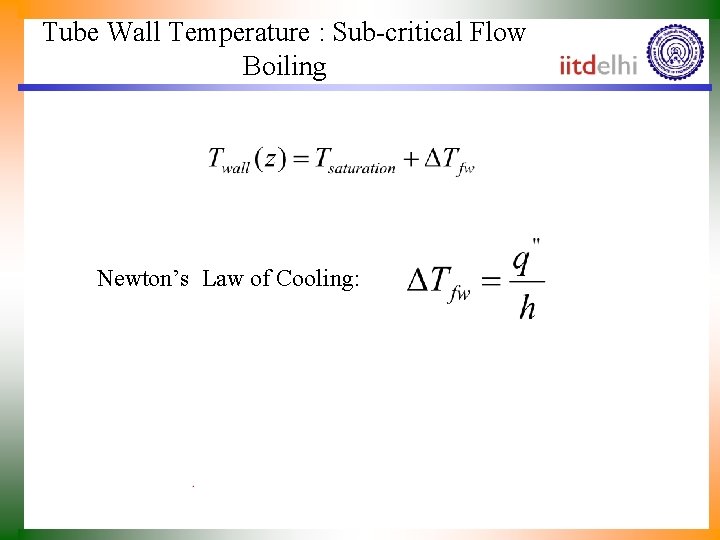
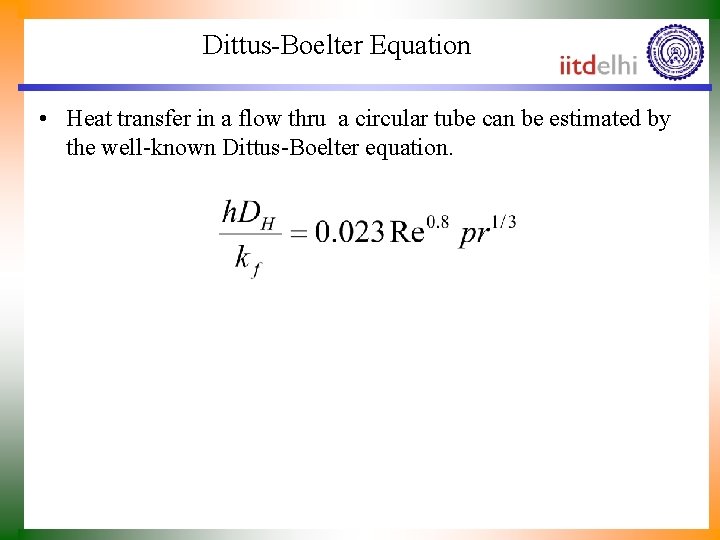
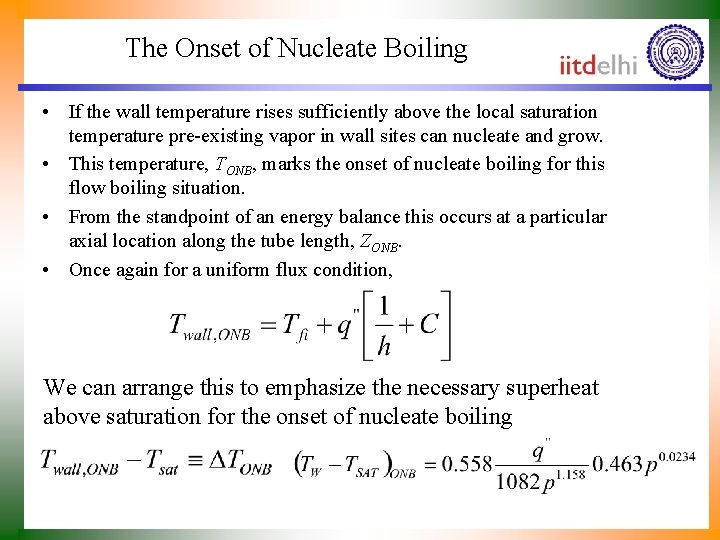
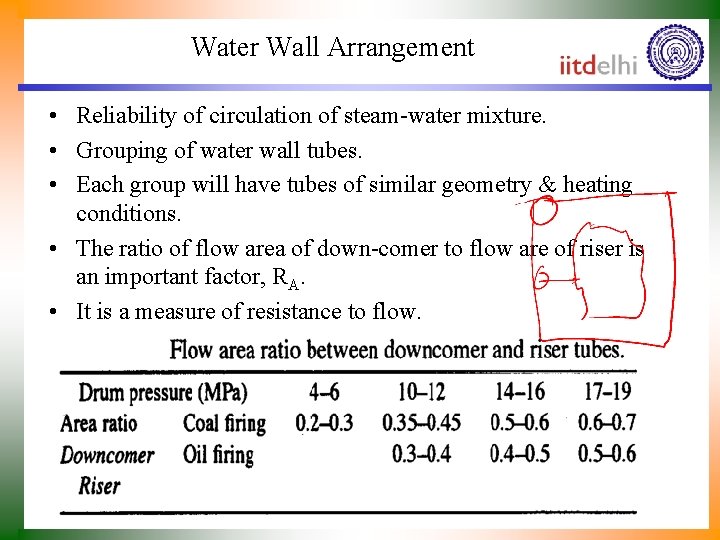
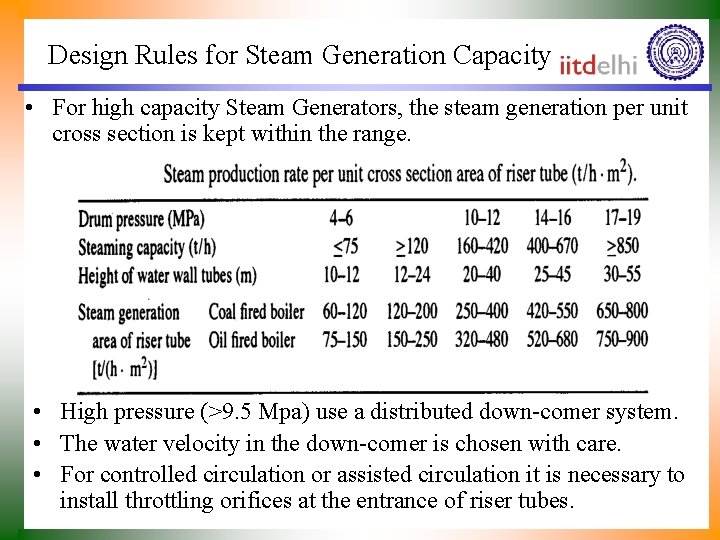
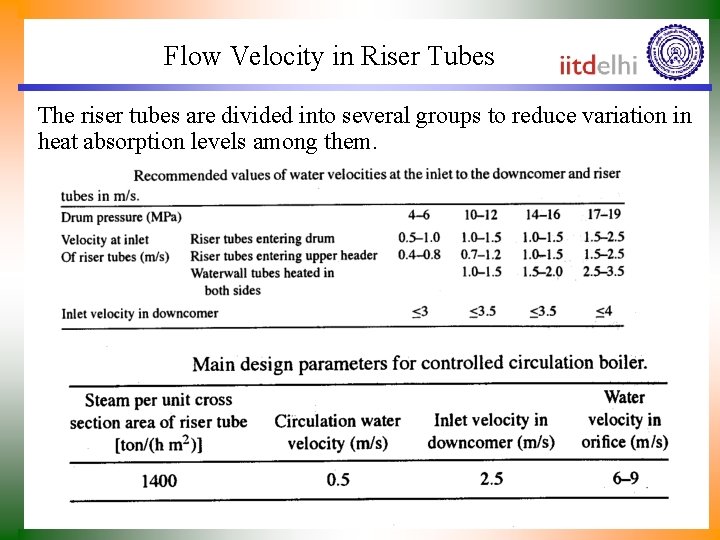
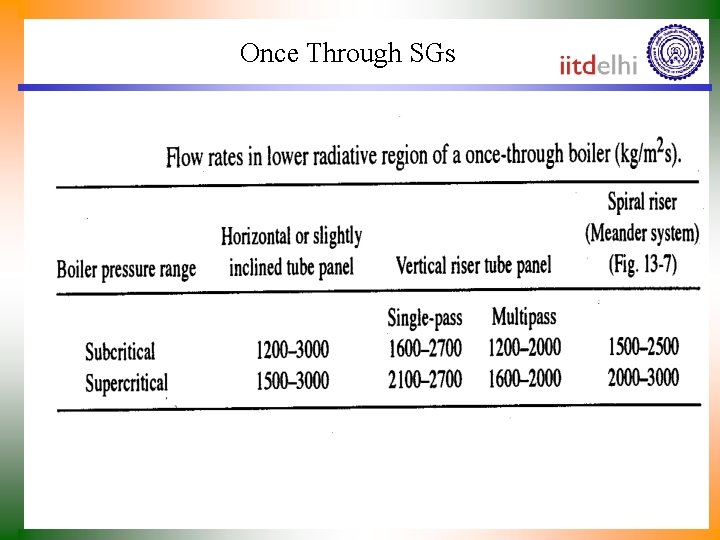
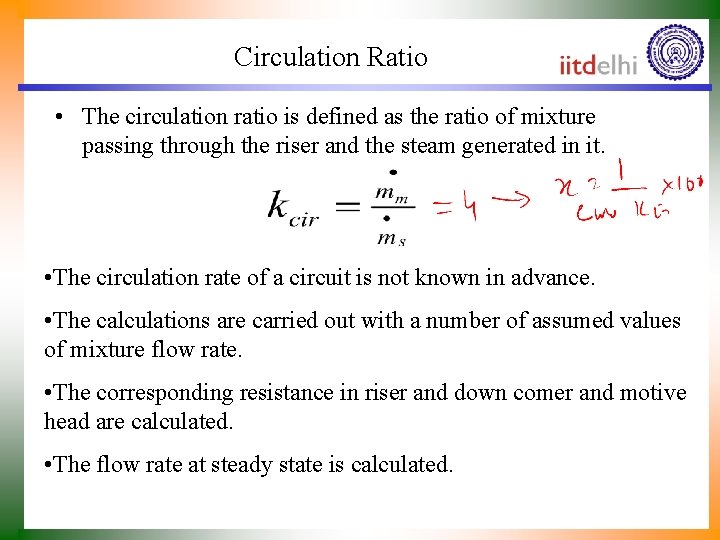
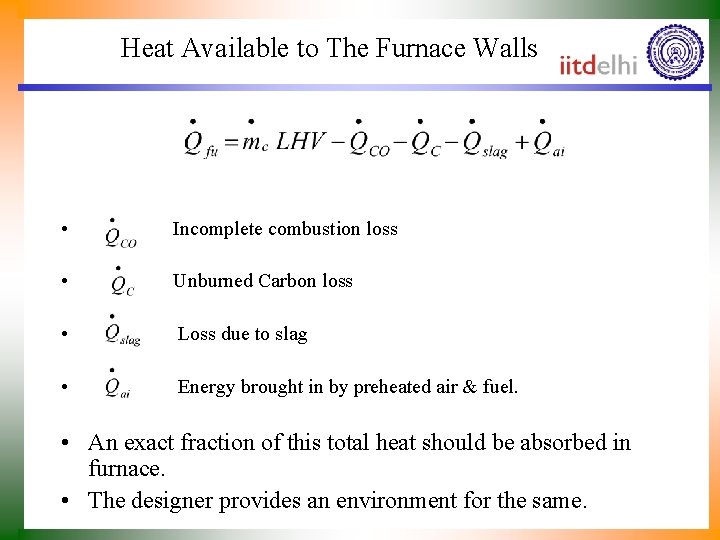
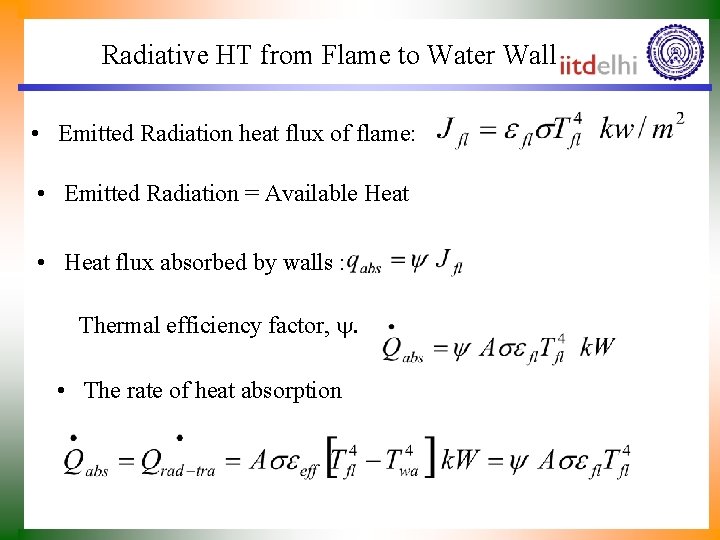
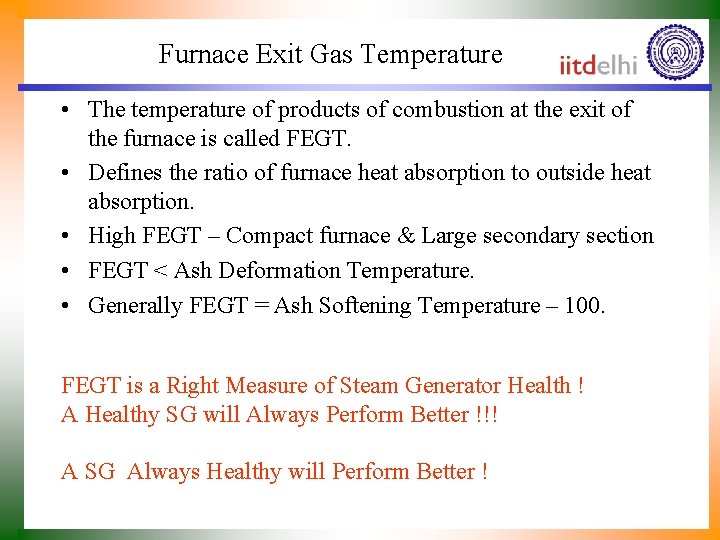
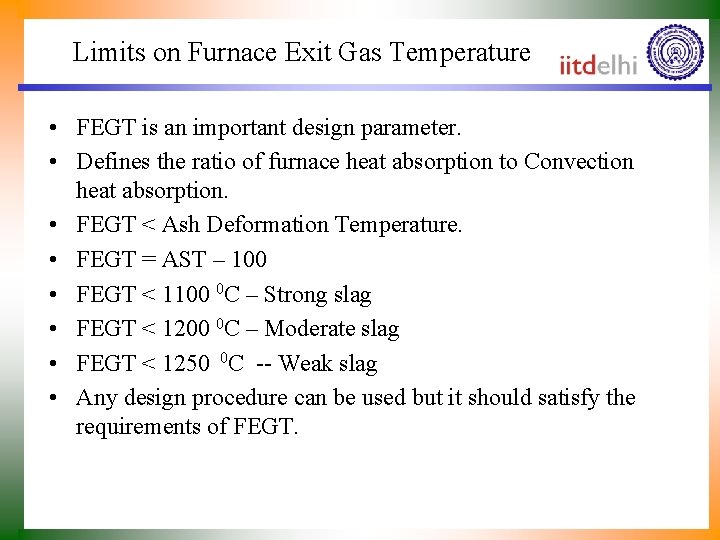
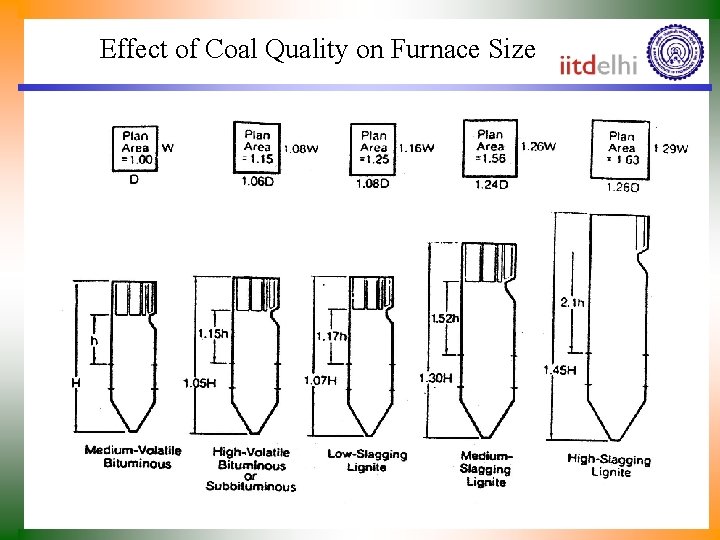
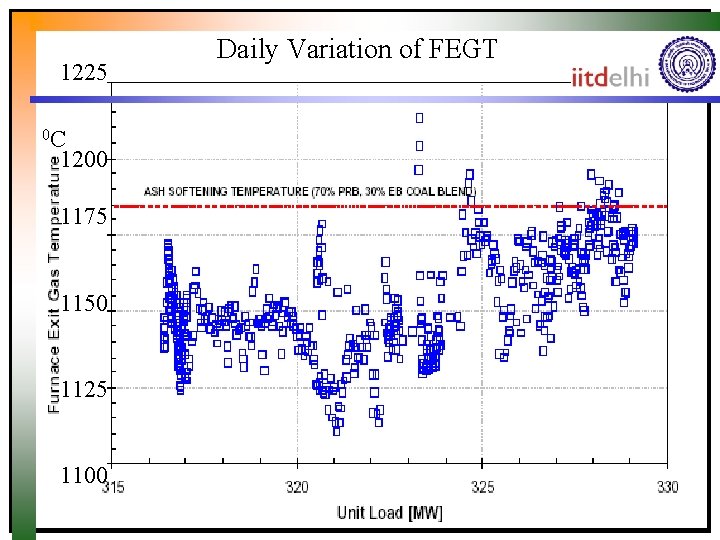
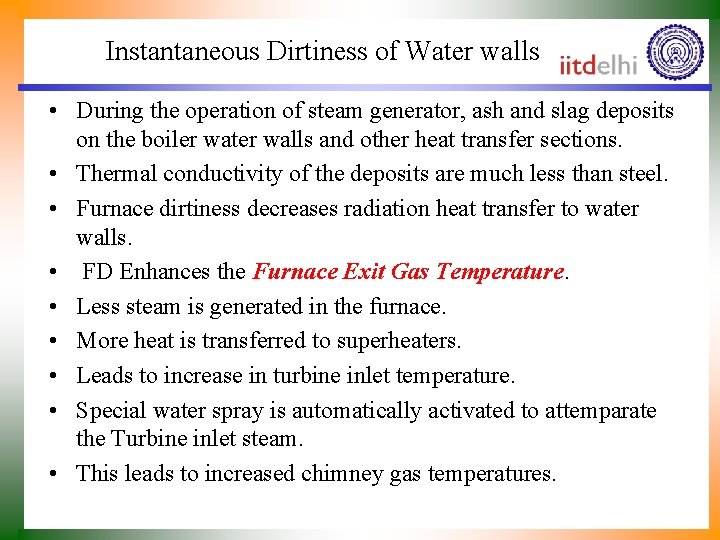
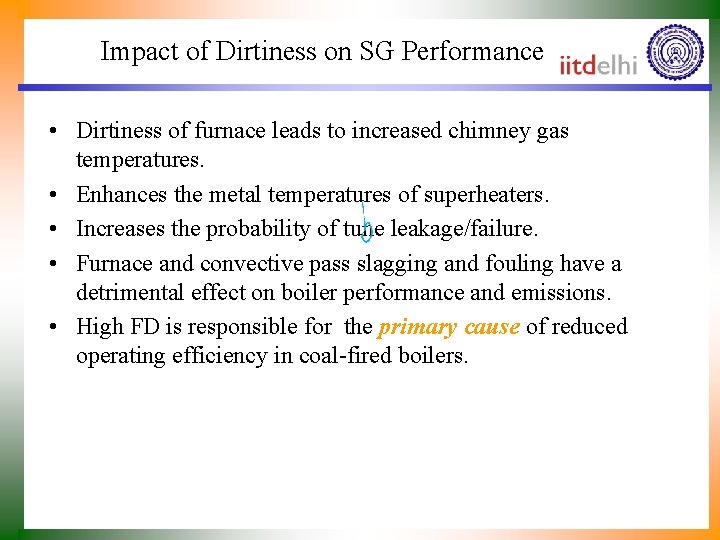
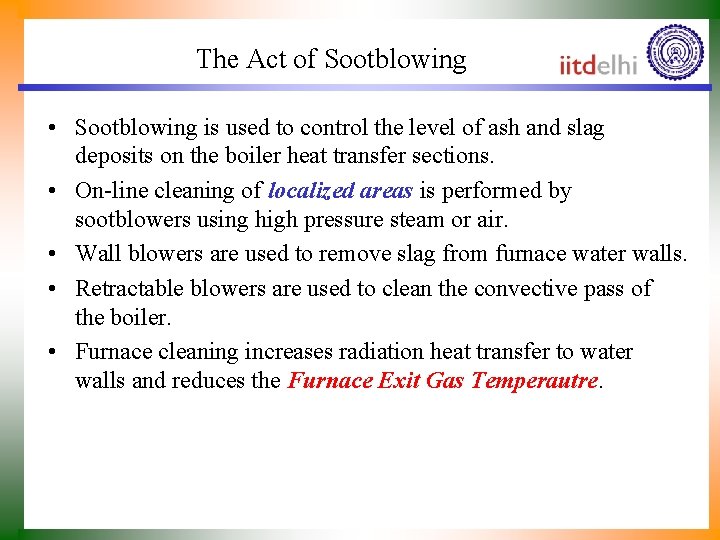
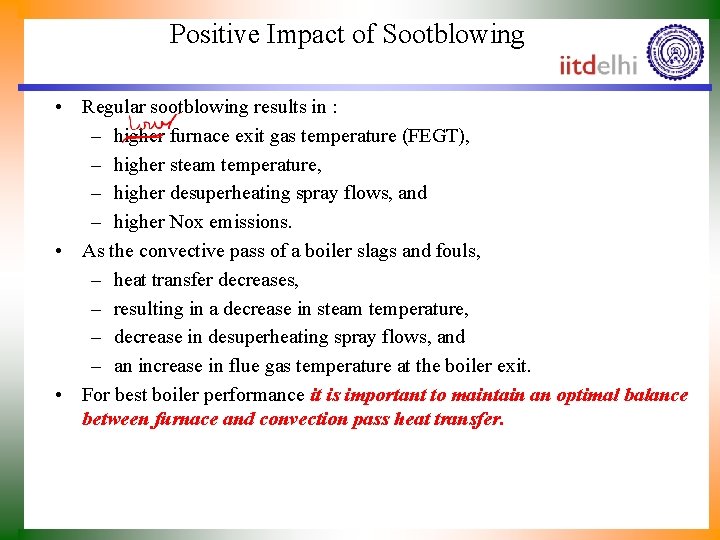
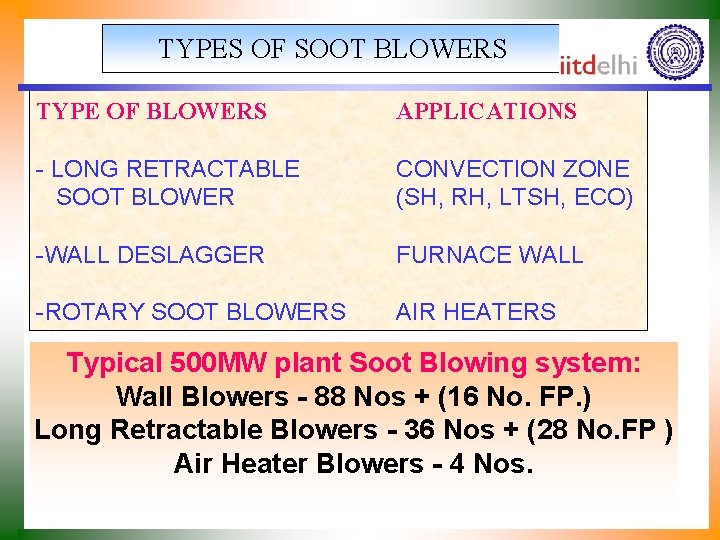
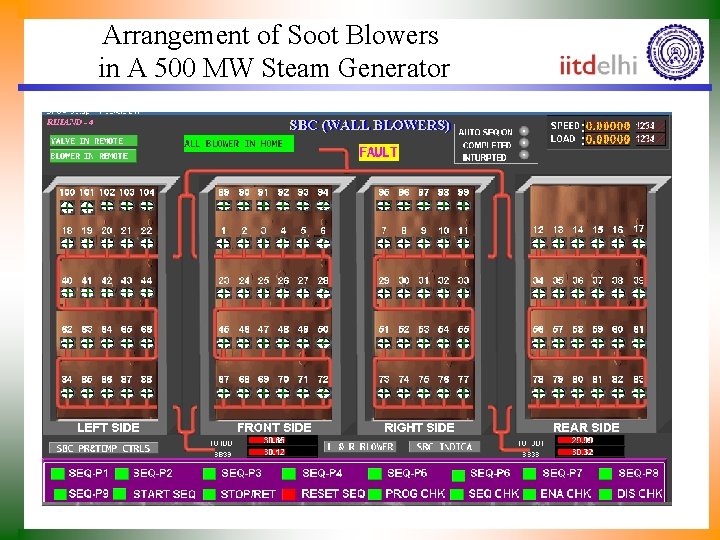
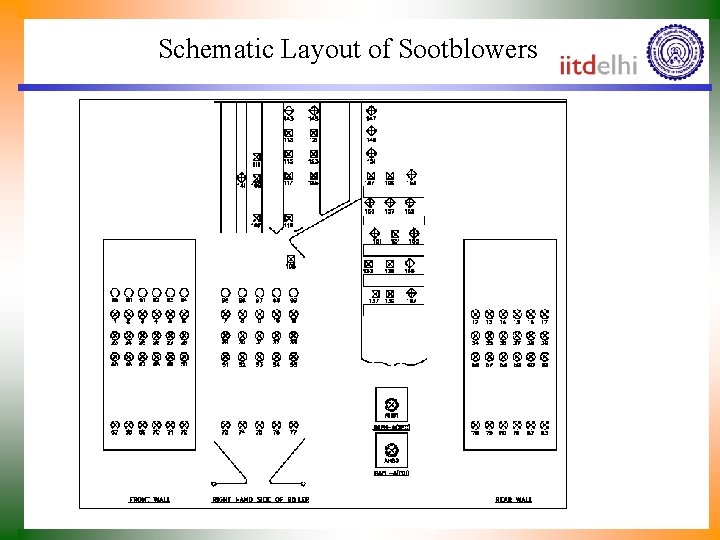
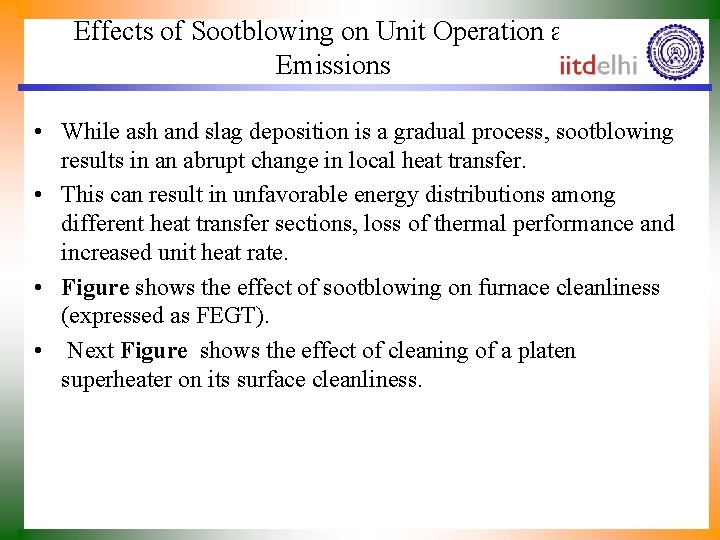
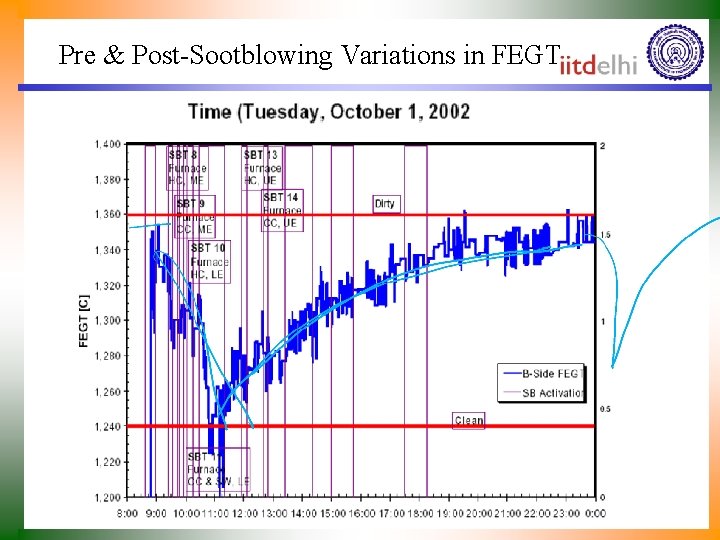
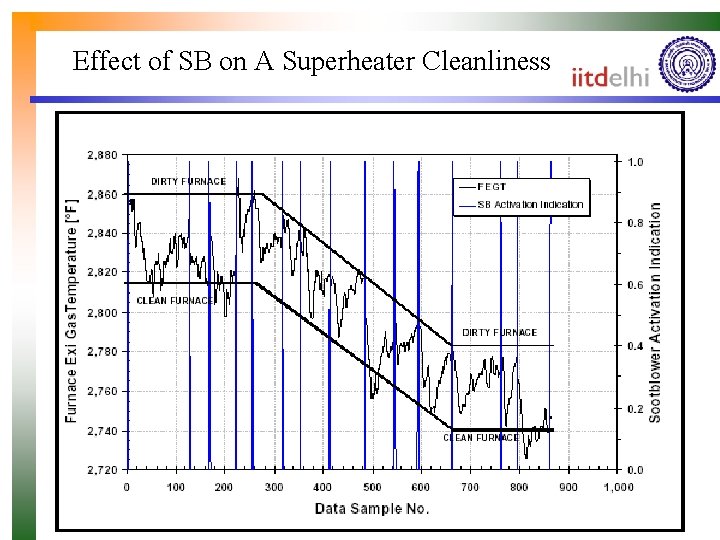
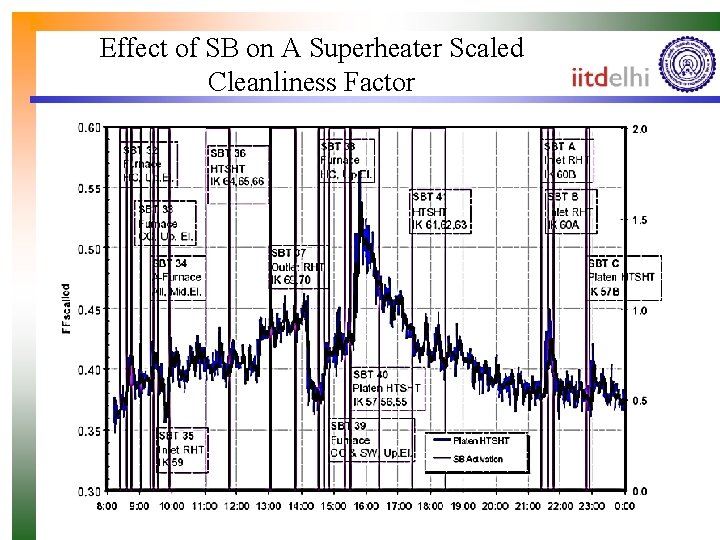
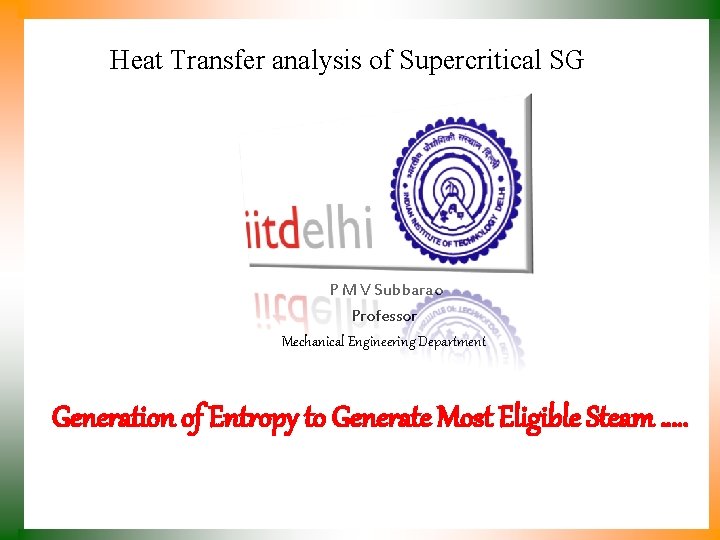
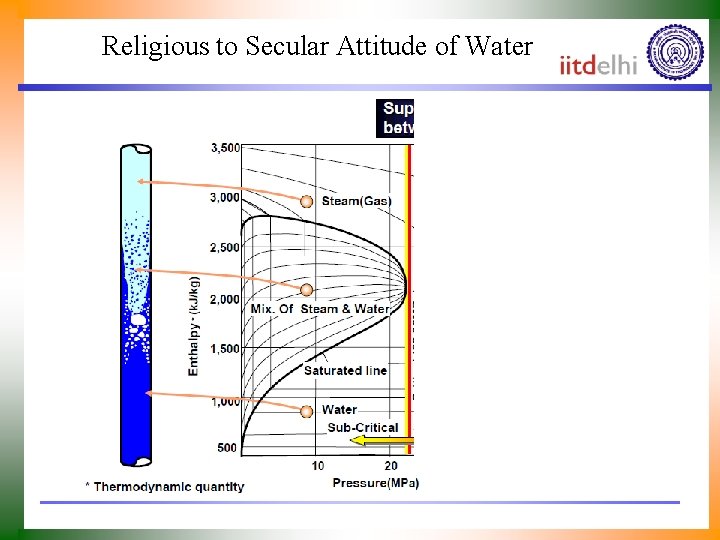
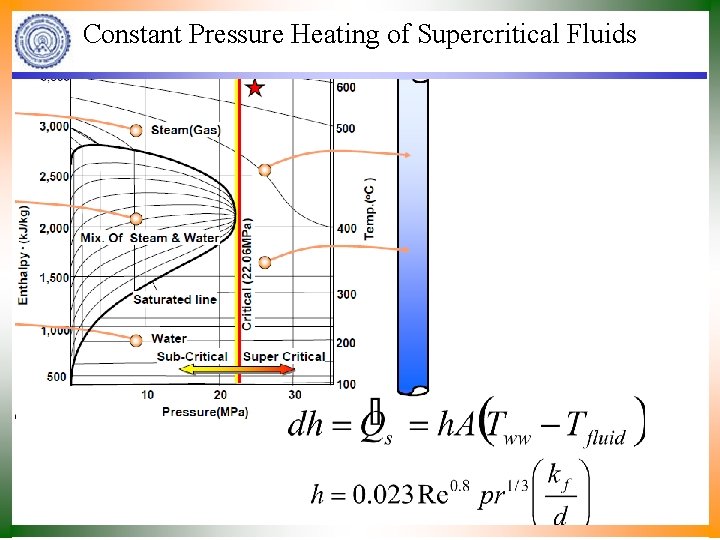
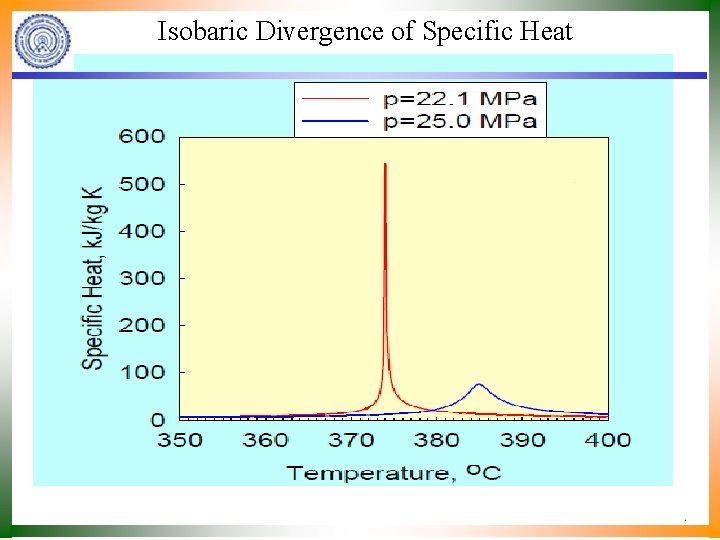
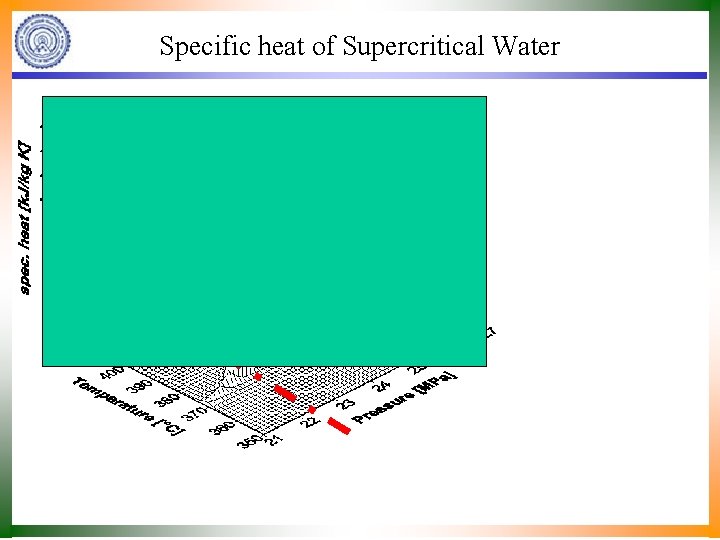
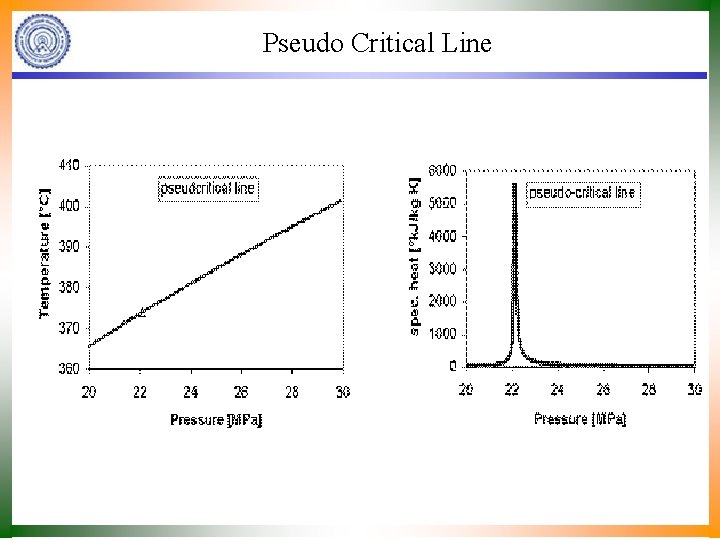
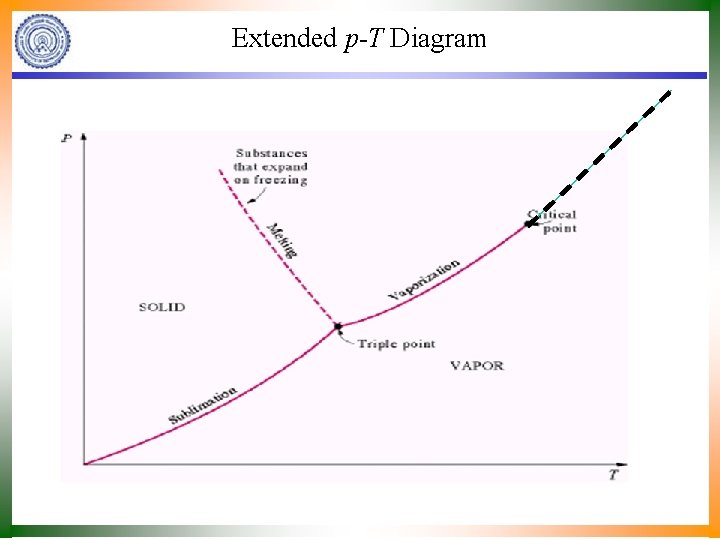
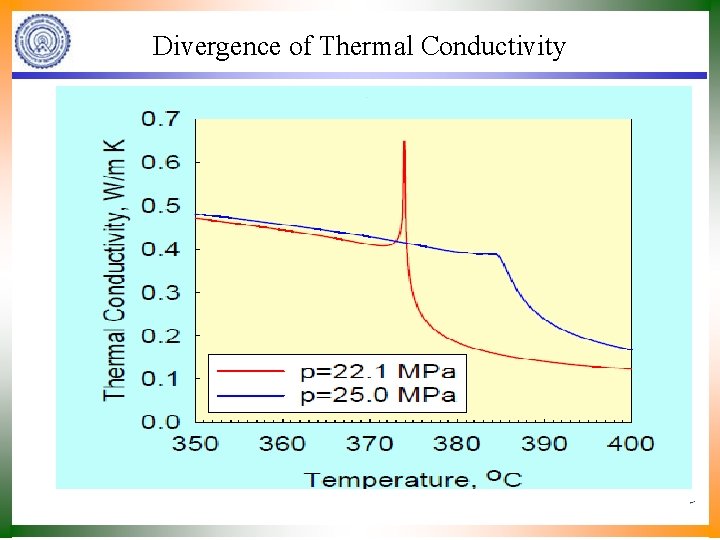
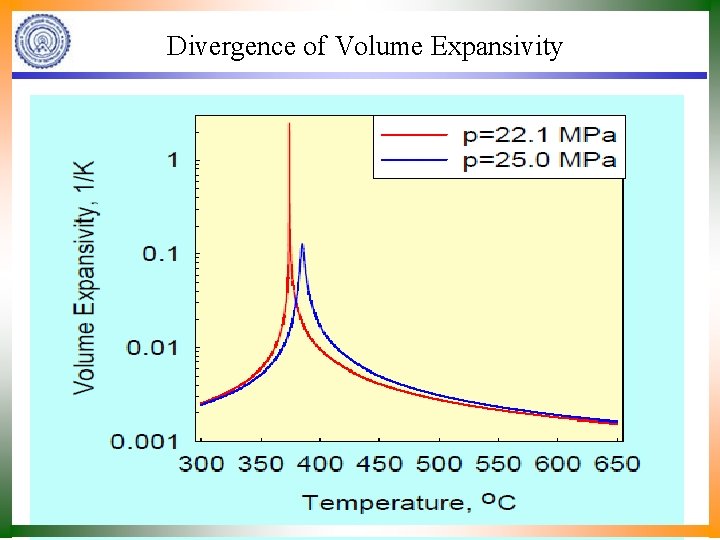
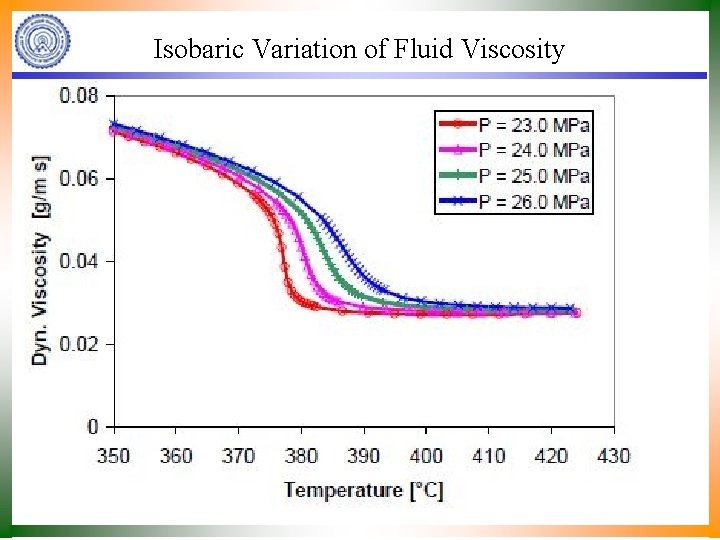
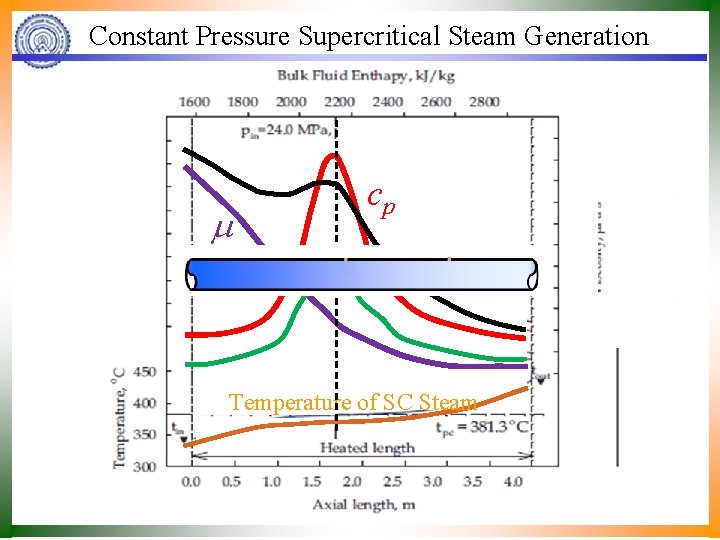
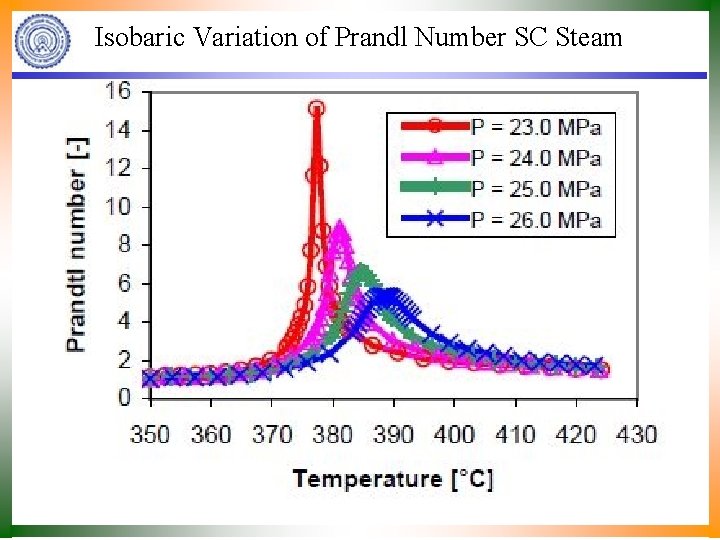
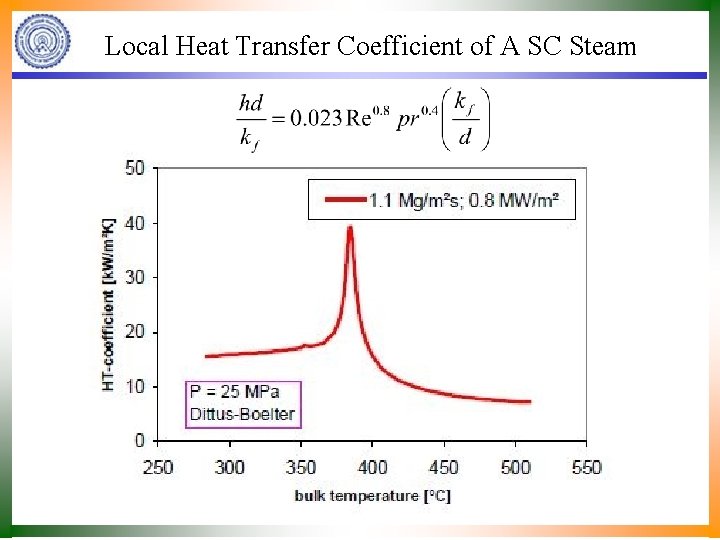
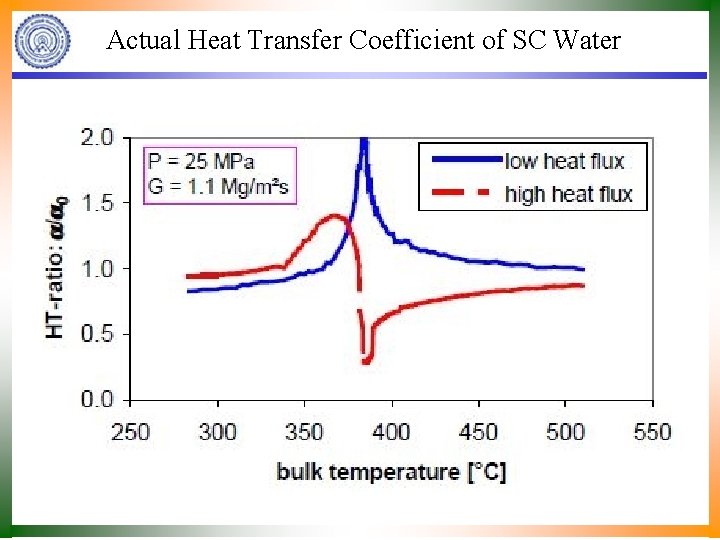
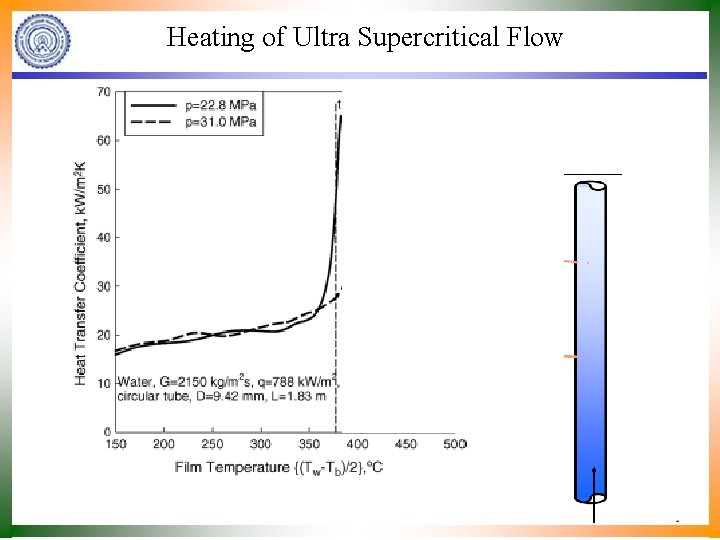
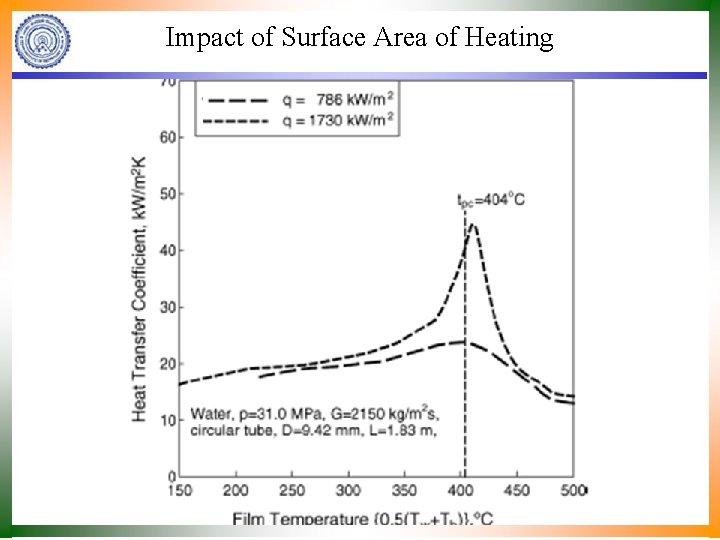
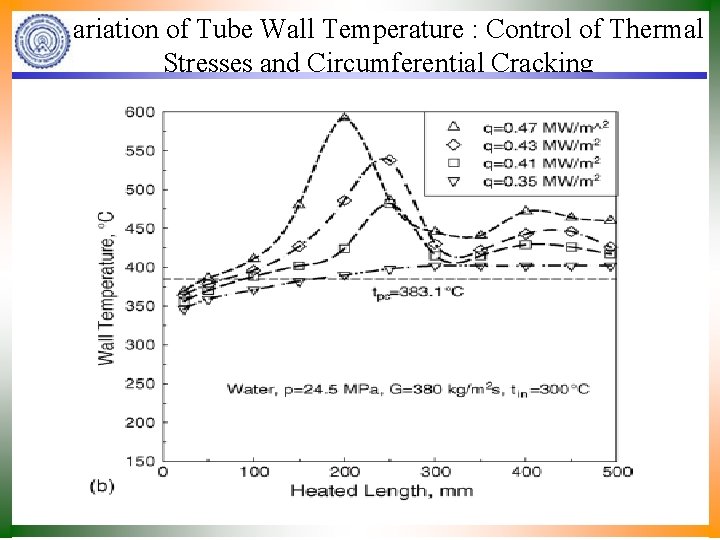
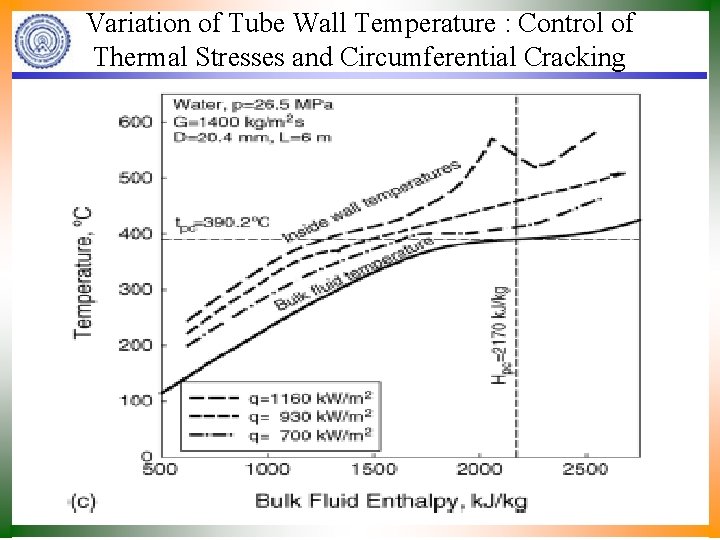
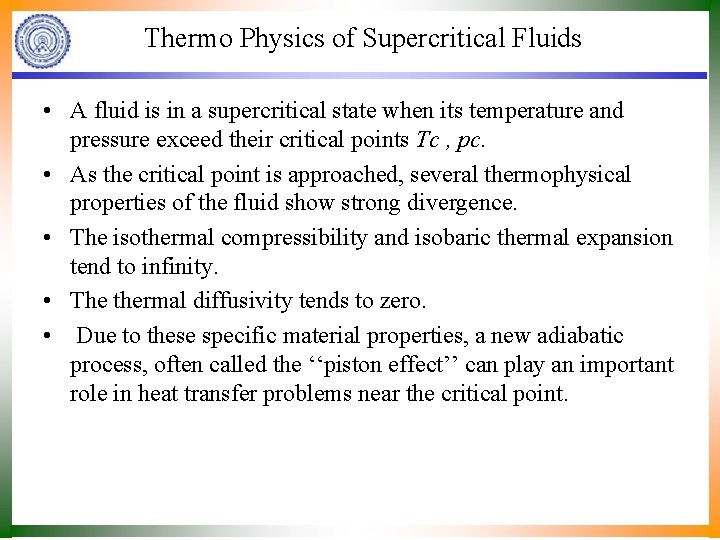
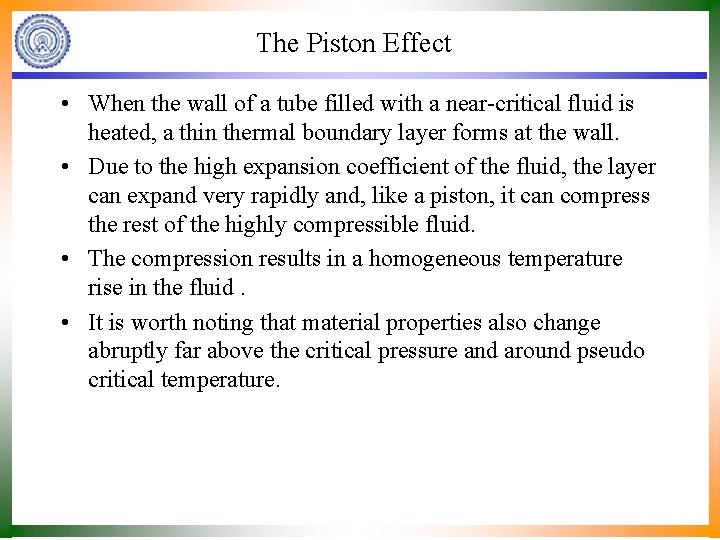
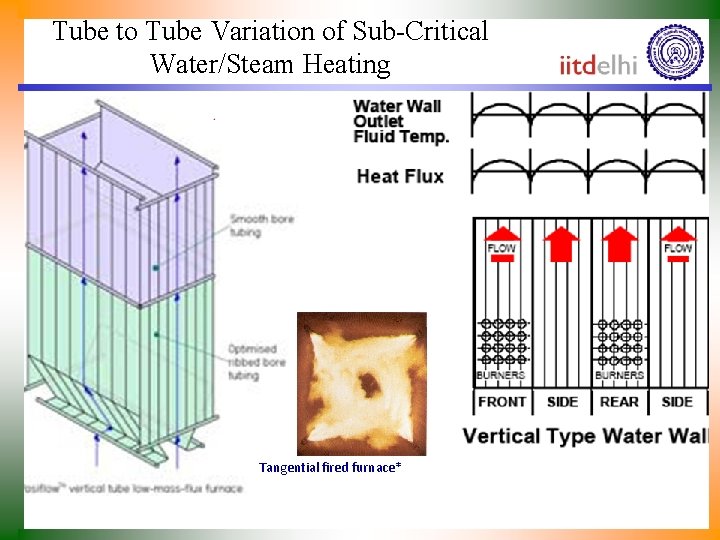
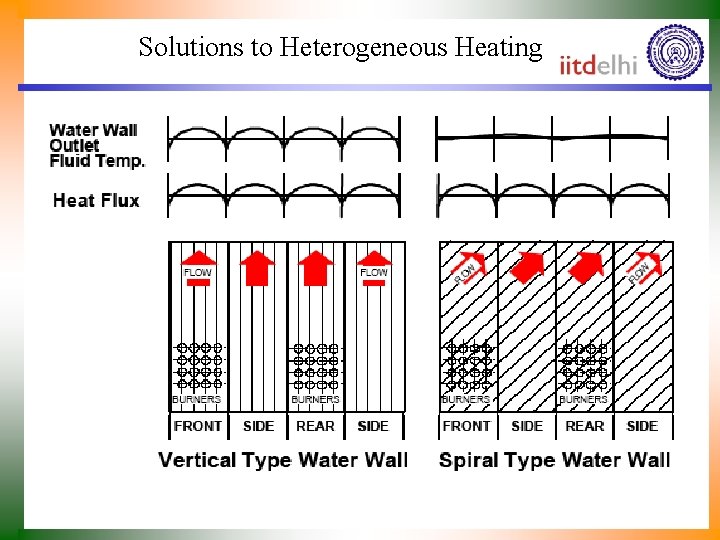
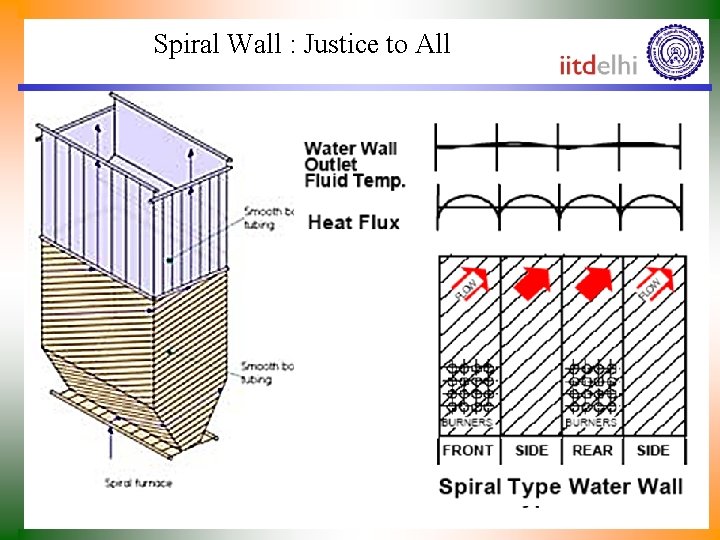
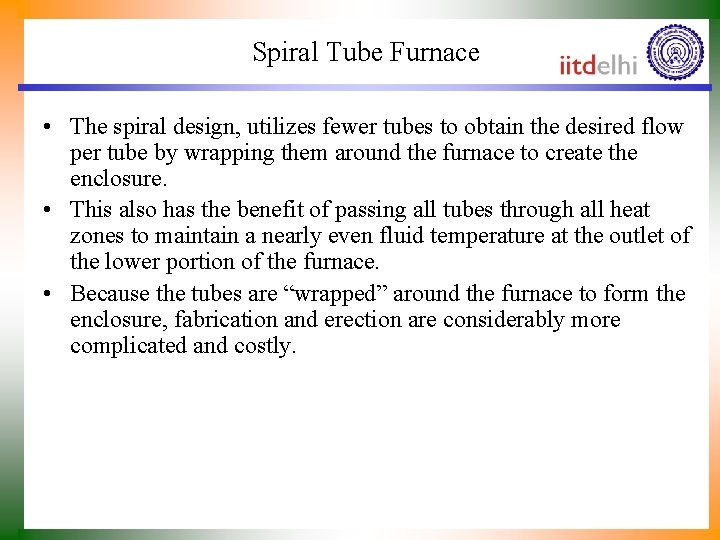
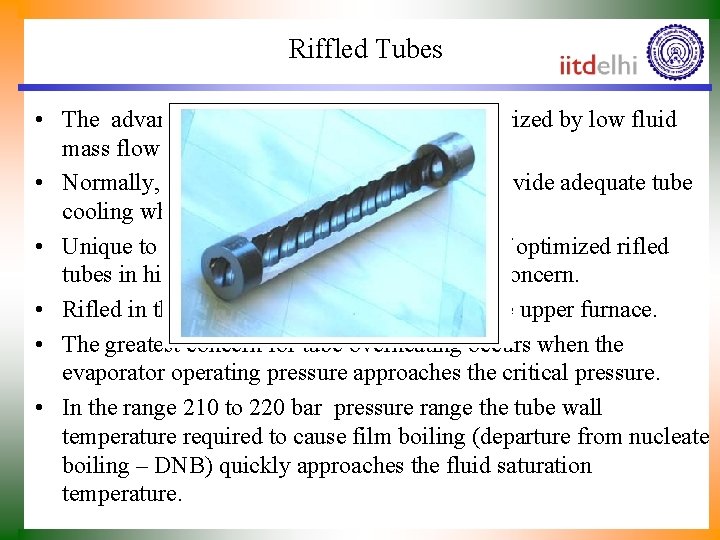
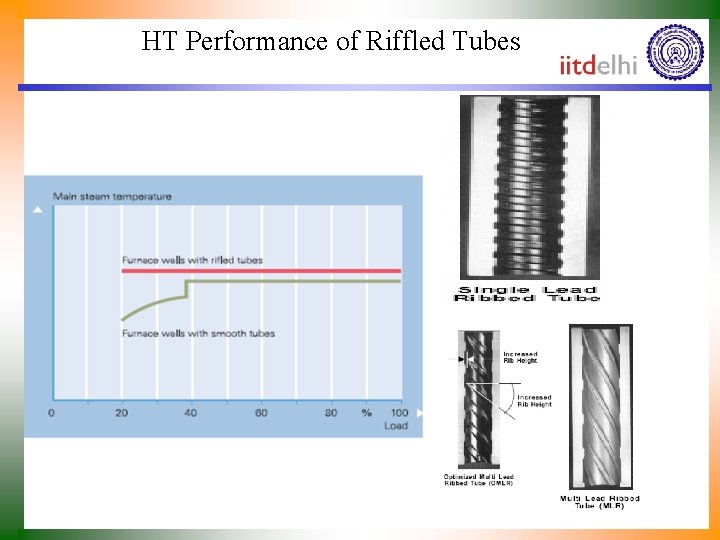
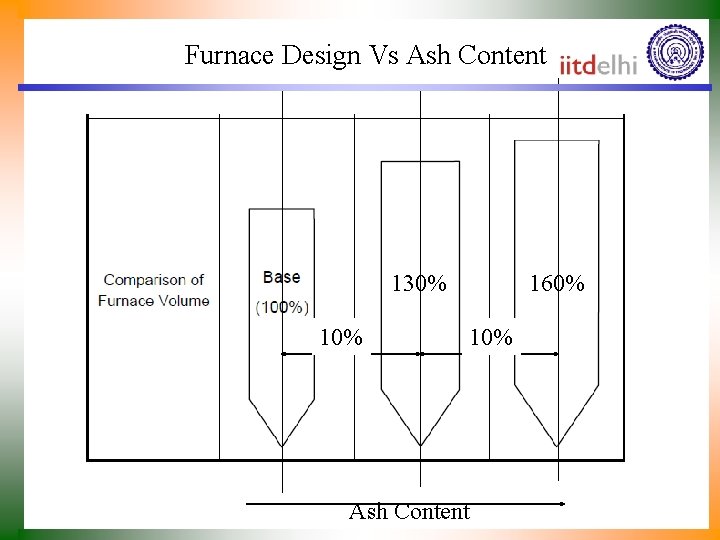
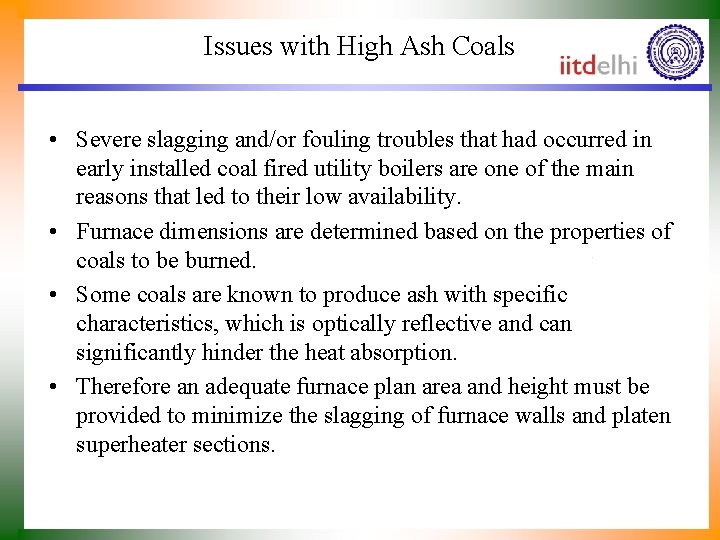
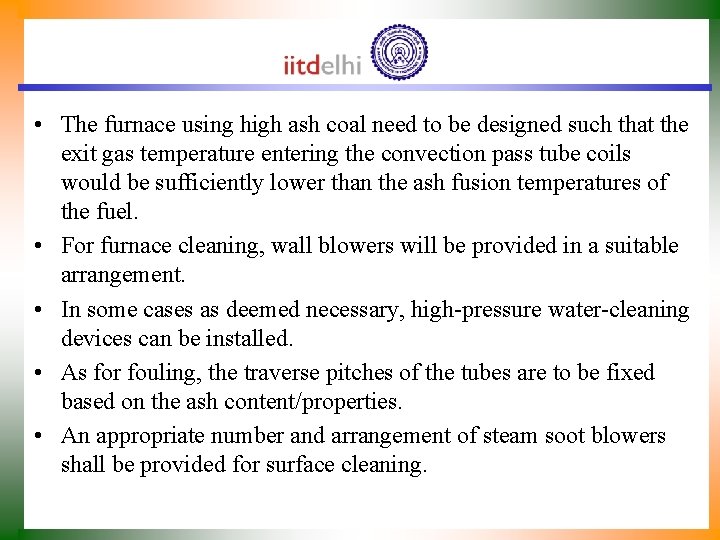
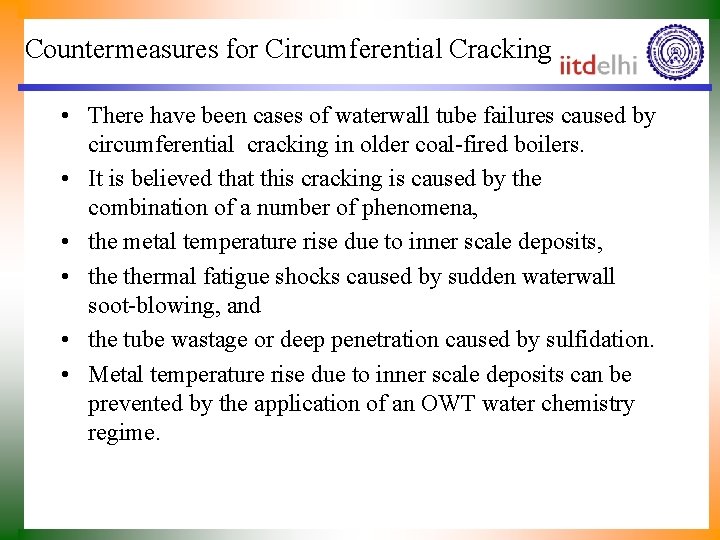
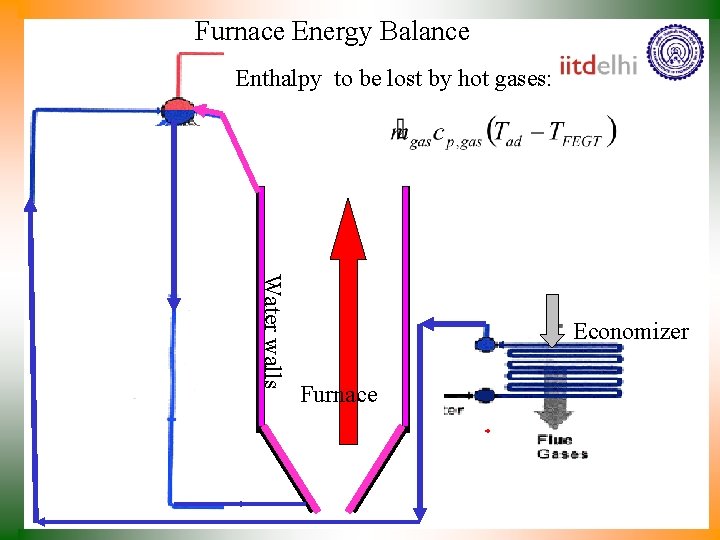
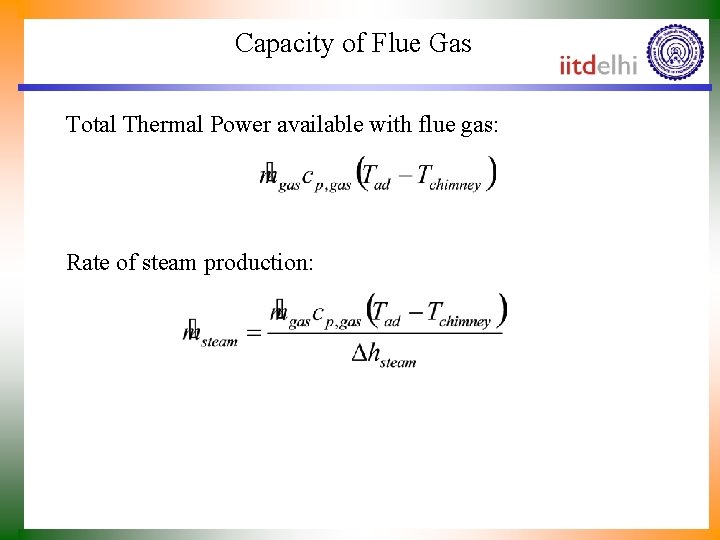
- Slides: 77
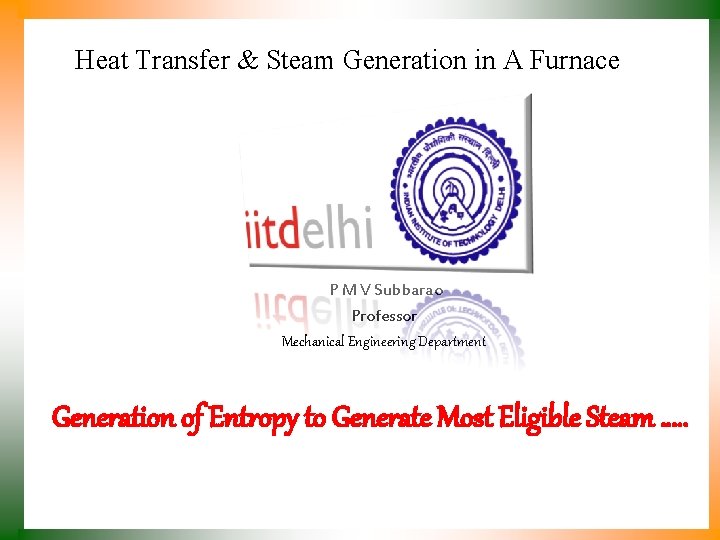
Heat Transfer & Steam Generation in A Furnace P M V Subbarao Professor Mechanical Engineering Department Generation of Entropy to Generate Most Eligible Steam …. .
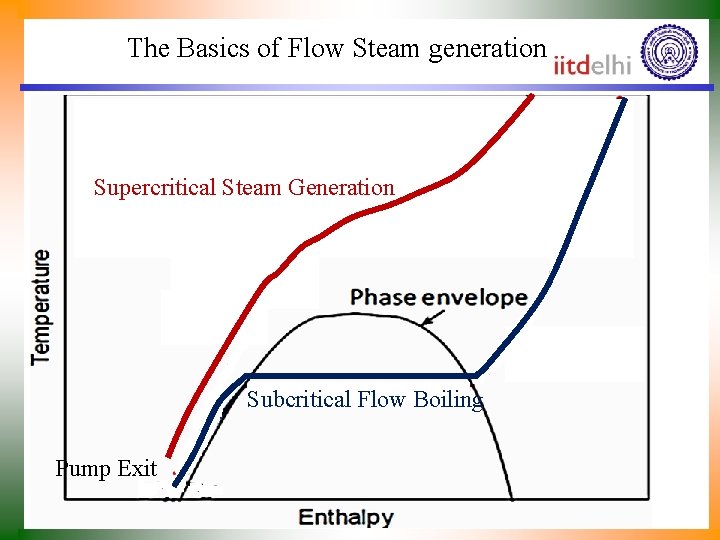
The Basics of Flow Steam generation Supercritical Steam Generation Subcritical Flow Boiling Pump Exit
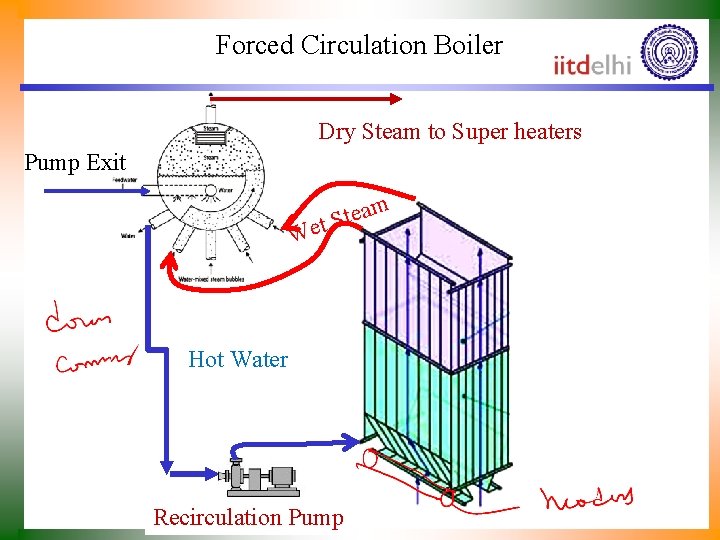
Forced Circulation Boiler Dry Steam to Super heaters Pump Exit m a e t et S W Hot Water Recirculation Pump
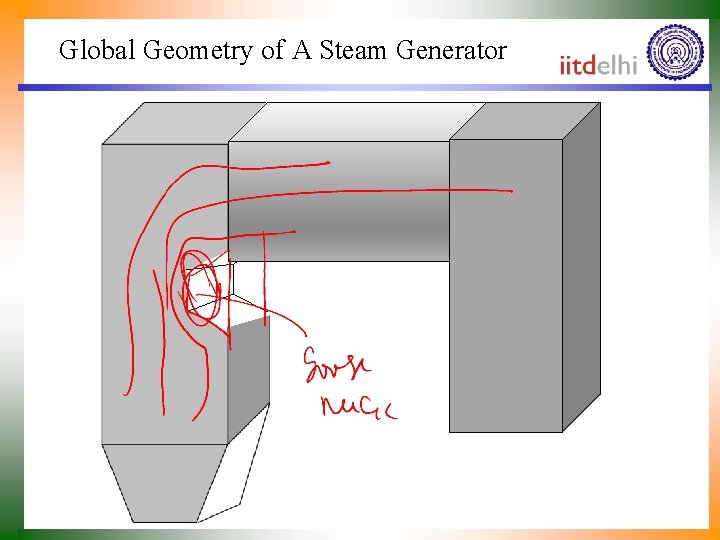
Global Geometry of A Steam Generator
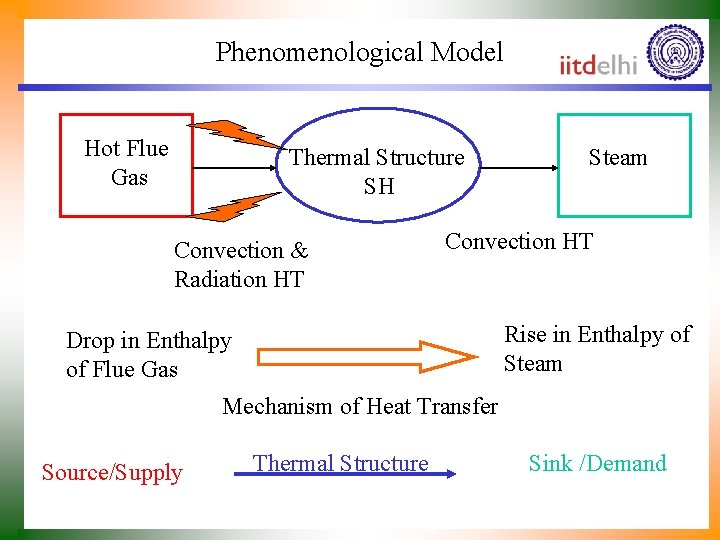
Phenomenological Model Hot Flue Gas Thermal Structure SH Convection & Radiation HT Steam Convection HT Rise in Enthalpy of Steam Drop in Enthalpy of Flue Gas Mechanism of Heat Transfer Source/Supply Thermal Structure Sink /Demand
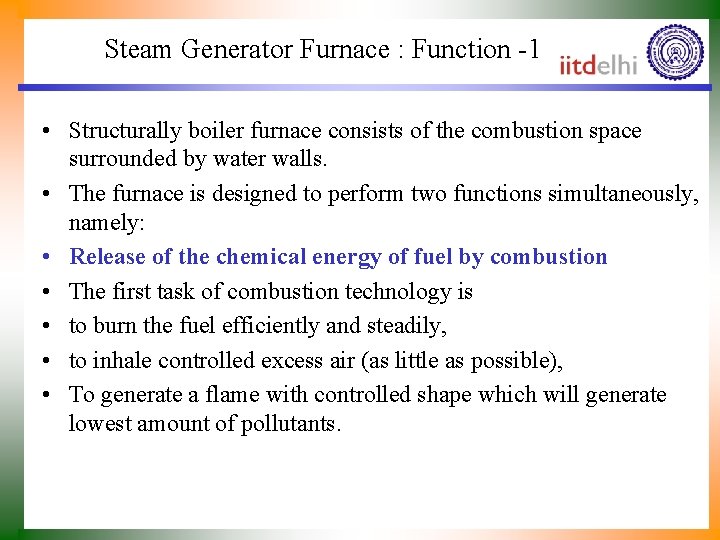
Steam Generator Furnace : Function -1 • Structurally boiler furnace consists of the combustion space surrounded by water walls. • The furnace is designed to perform two functions simultaneously, namely: • Release of the chemical energy of fuel by combustion • The first task of combustion technology is • to burn the fuel efficiently and steadily, • to inhale controlled excess air (as little as possible), • To generate a flame with controlled shape which will generate lowest amount of pollutants.
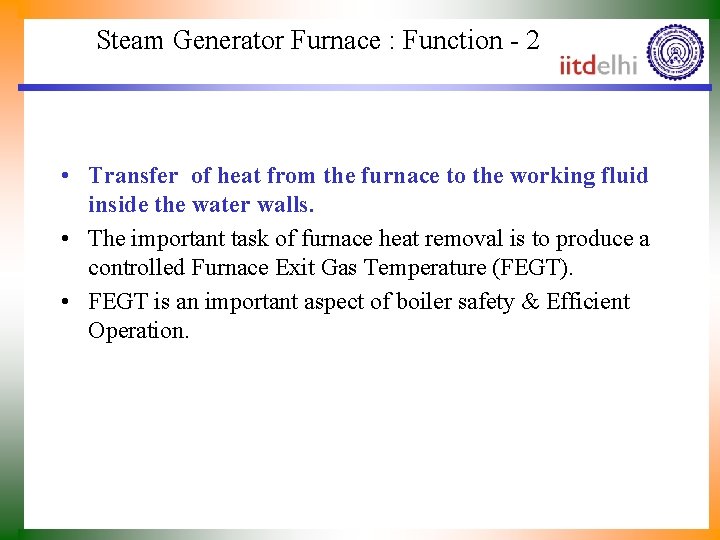
Steam Generator Furnace : Function - 2 • Transfer of heat from the furnace to the working fluid inside the water walls. • The important task of furnace heat removal is to produce a controlled Furnace Exit Gas Temperature (FEGT). • FEGT is an important aspect of boiler safety & Efficient Operation.
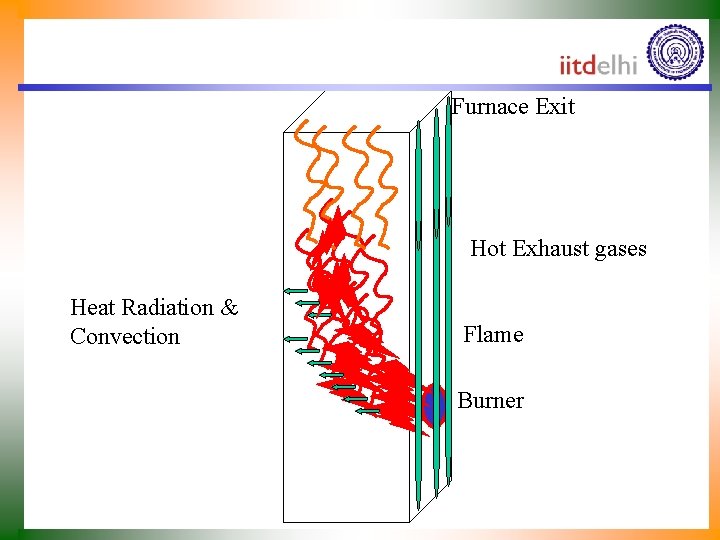
Furnace Exit Hot Exhaust gases Heat Radiation & Convection Flame Burner
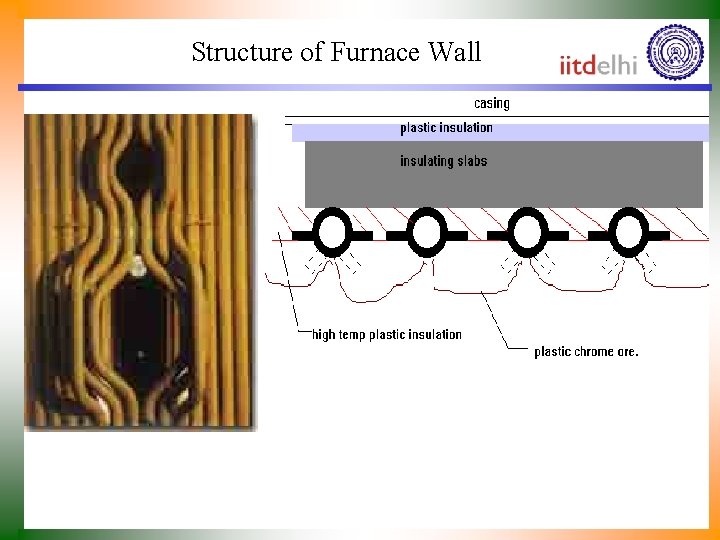
Structure of Furnace Wall
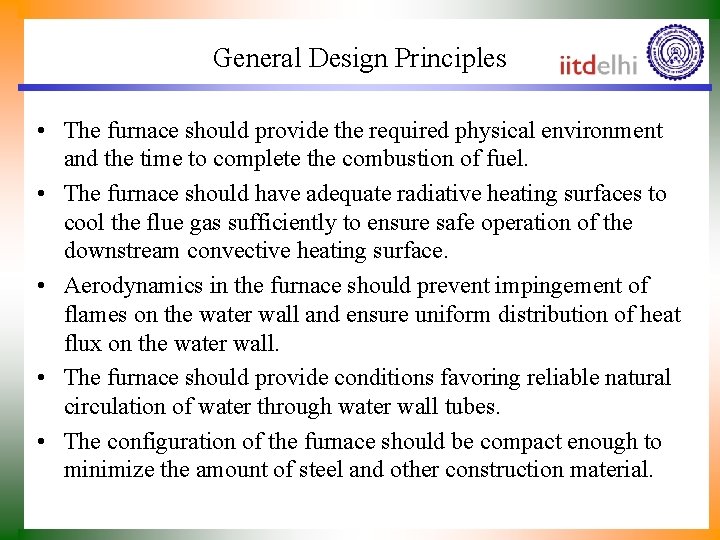
General Design Principles • The furnace should provide the required physical environment and the time to complete the combustion of fuel. • The furnace should have adequate radiative heating surfaces to cool the flue gas sufficiently to ensure safe operation of the downstream convective heating surface. • Aerodynamics in the furnace should prevent impingement of flames on the water wall and ensure uniform distribution of heat flux on the water wall. • The furnace should provide conditions favoring reliable natural circulation of water through water wall tubes. • The configuration of the furnace should be compact enough to minimize the amount of steel and other construction material.
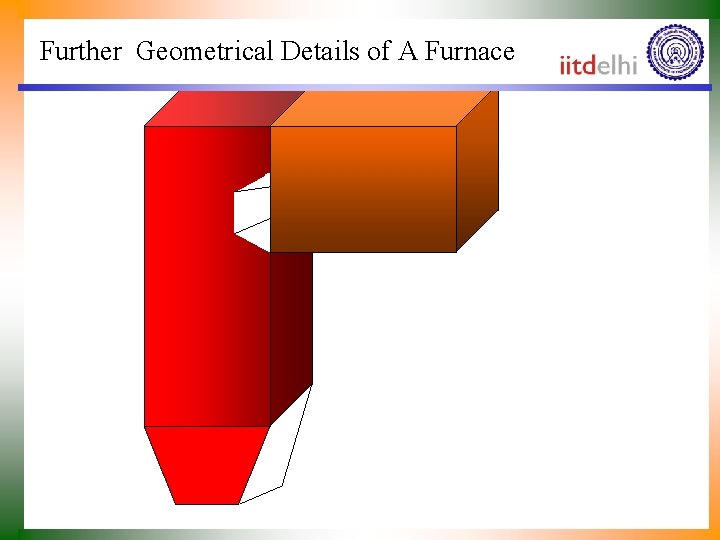
Further Geometrical Details of A Furnace
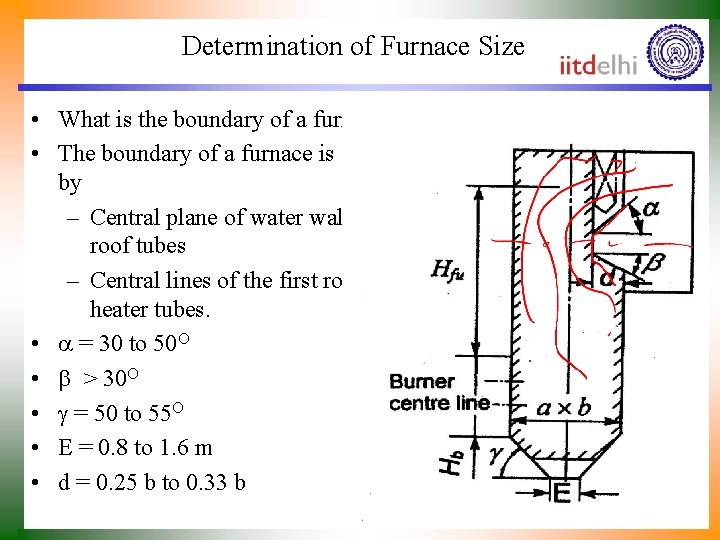
Determination of Furnace Size • What is the boundary of a furnace? • The boundary of a furnace is defined by – Central plane of water wall and roof tubes – Central lines of the first row super heater tubes. • = 30 to 50 O • > 30 O • = 50 to 55 O • E = 0. 8 to 1. 6 m • d = 0. 25 b to 0. 33 b
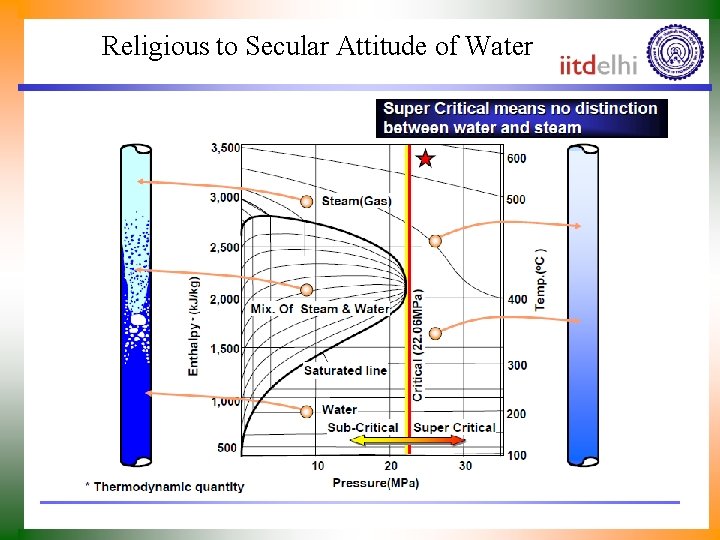
Religious to Secular Attitude of Water
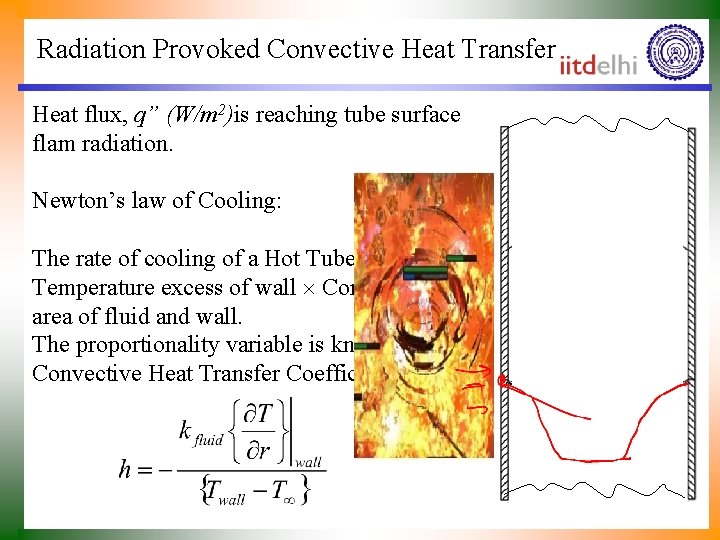
Radiation Provoked Convective Heat Transfer Heat flux, q” (W/m 2)is reaching tube surface flam radiation. Newton’s law of Cooling: The rate of cooling of a Hot Tube Wall Temperature excess of wall Contact Surface area of fluid and wall. The proportionality variable is known as Convective Heat Transfer Coefficient.
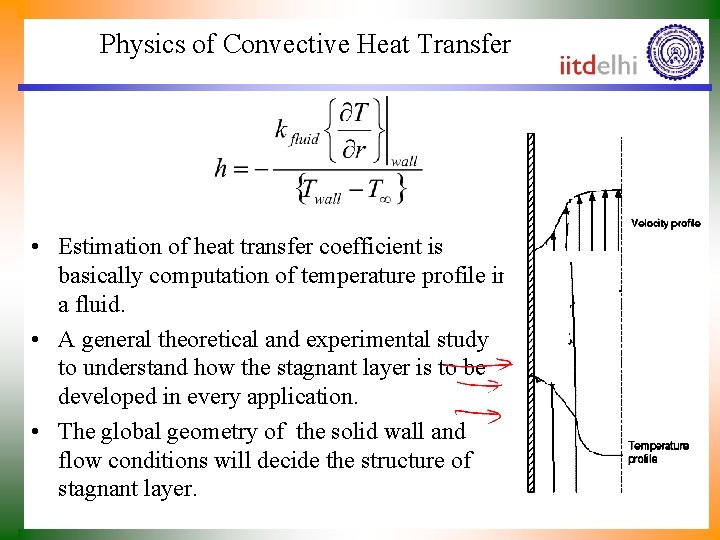
Physics of Convective Heat Transfer • Estimation of heat transfer coefficient is basically computation of temperature profile in a fluid. • A general theoretical and experimental study to understand how the stagnant layer is to be developed in every application. • The global geometry of the solid wall and flow conditions will decide the structure of stagnant layer.
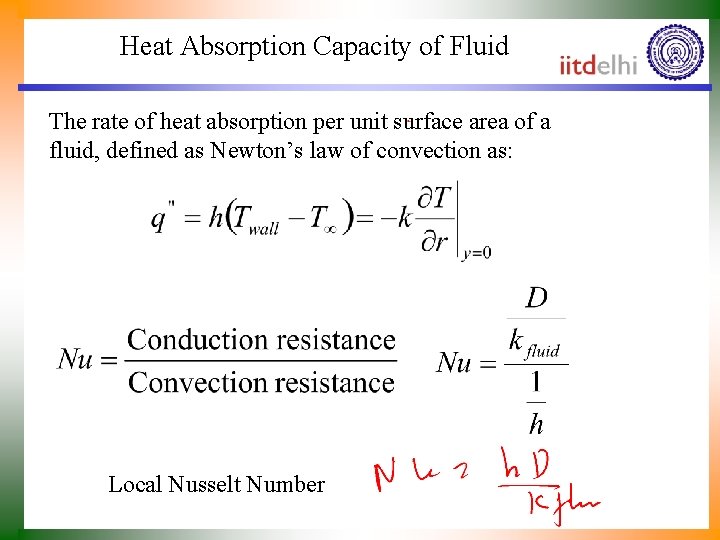
Heat Absorption Capacity of Fluid The rate of heat absorption per unit surface area of a fluid, defined as Newton’s law of convection as: Local Nusselt Number
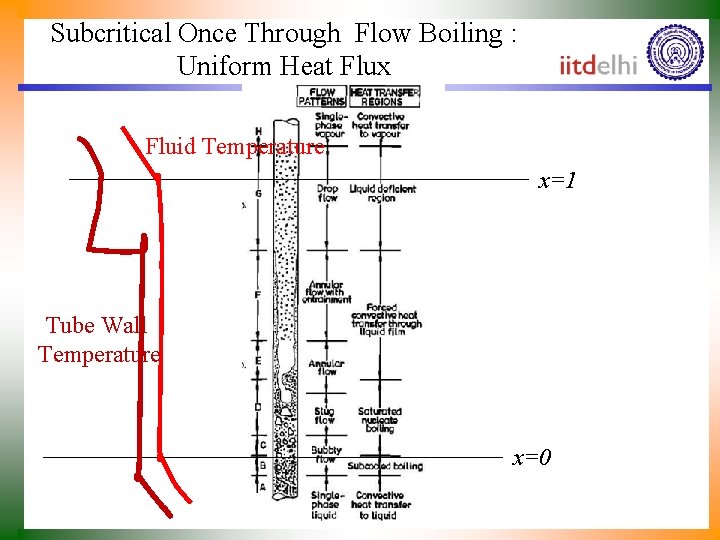
Subcritical Once Through Flow Boiling : Uniform Heat Flux Fluid Temperature x=1 Tube Wall Temperature x=0
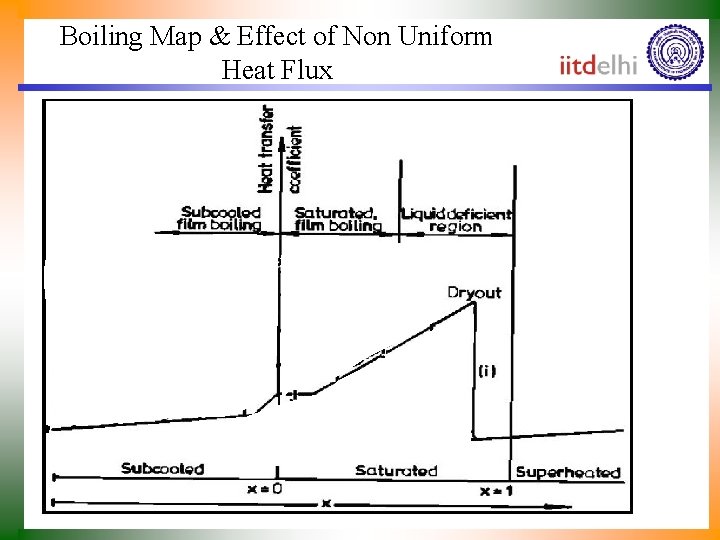
Boiling Map & Effect of Non Uniform Heat Flux
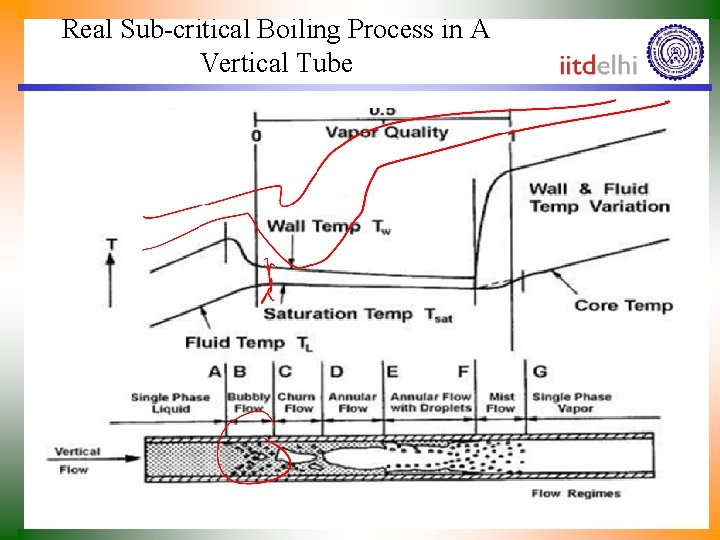
Real Sub-critical Boiling Process in A Vertical Tube
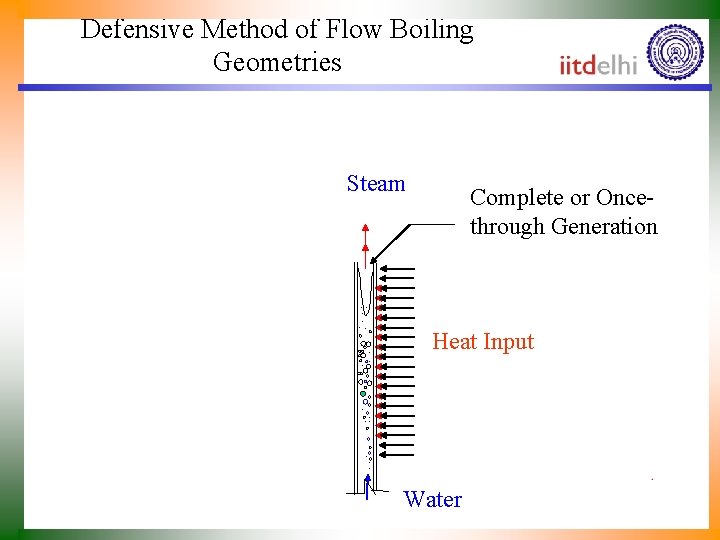
Defensive Method of Flow Boiling Geometries Steam Partial Steam Generation Water Heat Input Water Steam Complete or Oncethrough Generation Heat Input Water
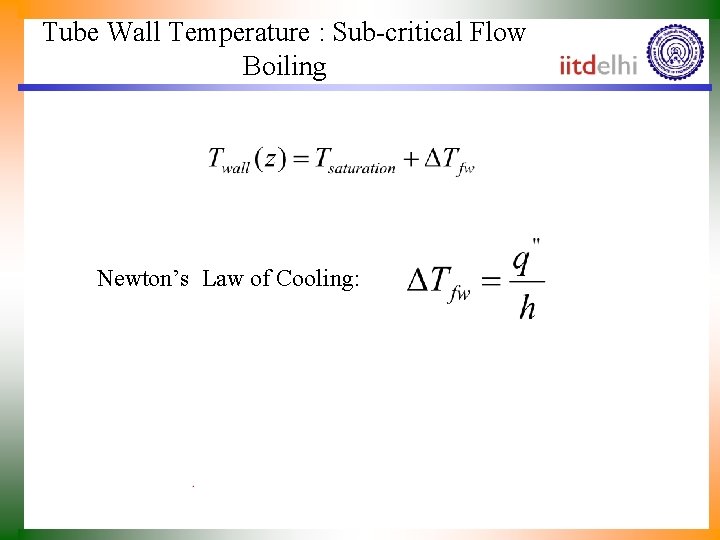
Tube Wall Temperature : Sub-critical Flow Boiling Newton’s Law of Cooling:
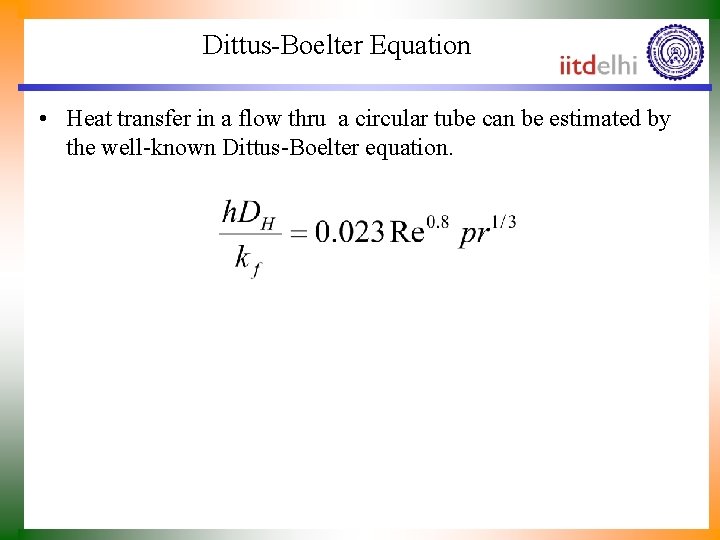
Dittus-Boelter Equation • Heat transfer in a flow thru a circular tube can be estimated by the well-known Dittus-Boelter equation.
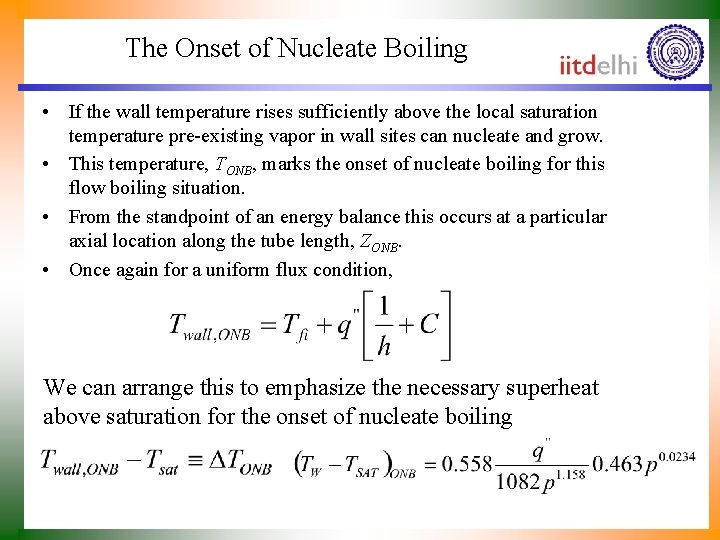
The Onset of Nucleate Boiling • If the wall temperature rises sufficiently above the local saturation temperature pre-existing vapor in wall sites can nucleate and grow. • This temperature, TONB, marks the onset of nucleate boiling for this flow boiling situation. • From the standpoint of an energy balance this occurs at a particular axial location along the tube length, ZONB. • Once again for a uniform flux condition, We can arrange this to emphasize the necessary superheat above saturation for the onset of nucleate boiling
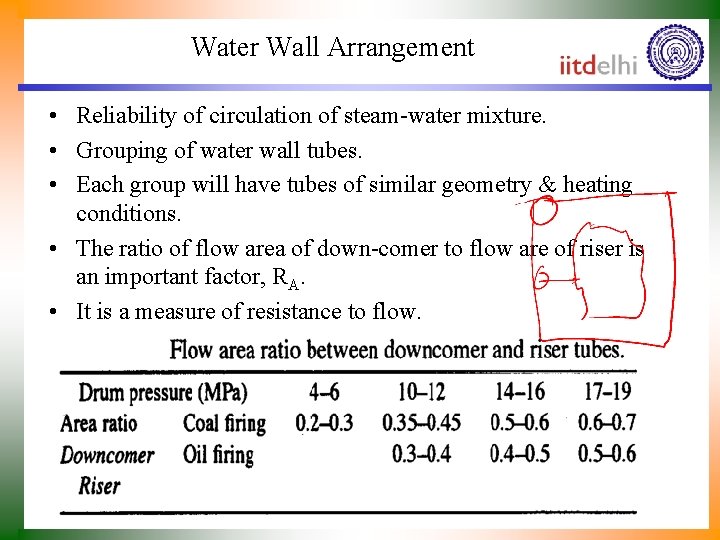
Water Wall Arrangement • Reliability of circulation of steam-water mixture. • Grouping of water wall tubes. • Each group will have tubes of similar geometry & heating conditions. • The ratio of flow area of down-comer to flow are of riser is an important factor, RA. • It is a measure of resistance to flow.
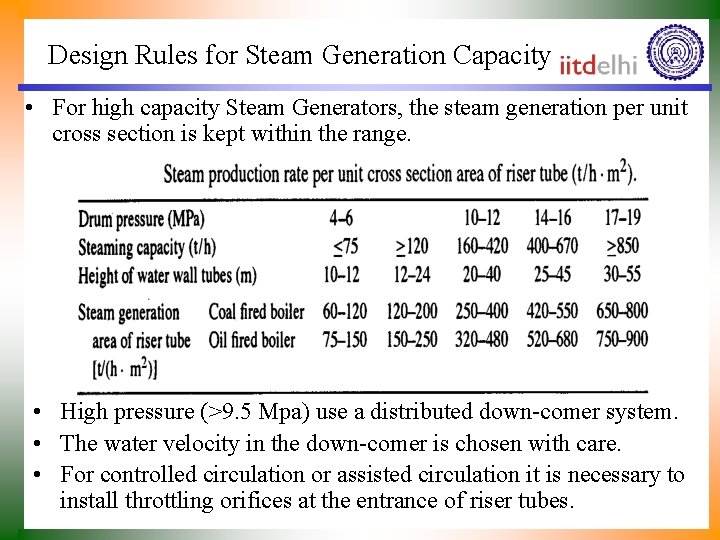
Design Rules for Steam Generation Capacity • For high capacity Steam Generators, the steam generation per unit cross section is kept within the range. • High pressure (>9. 5 Mpa) use a distributed down-comer system. • The water velocity in the down-comer is chosen with care. • For controlled circulation or assisted circulation it is necessary to install throttling orifices at the entrance of riser tubes.
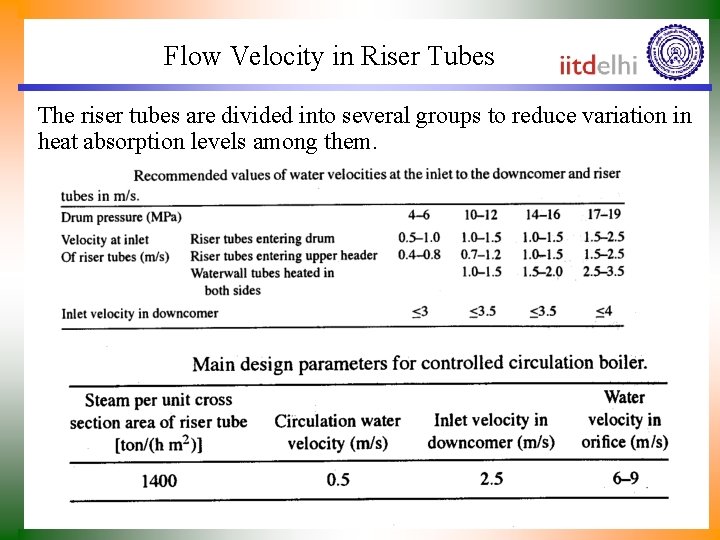
Flow Velocity in Riser Tubes The riser tubes are divided into several groups to reduce variation in heat absorption levels among them.
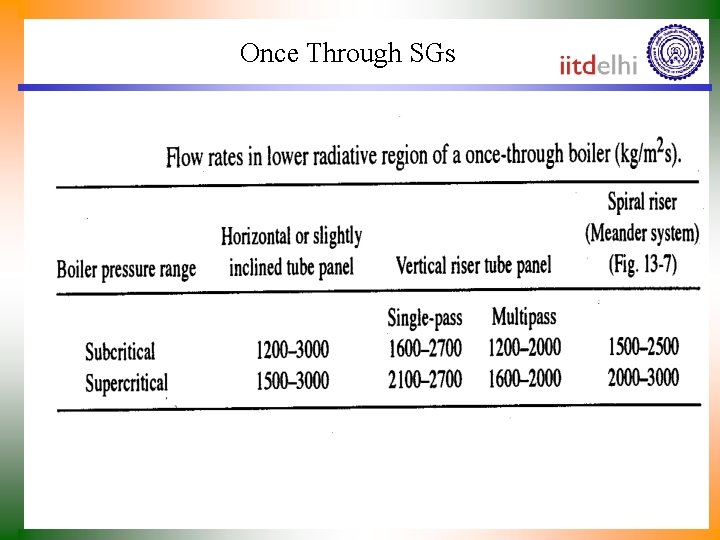
Once Through SGs
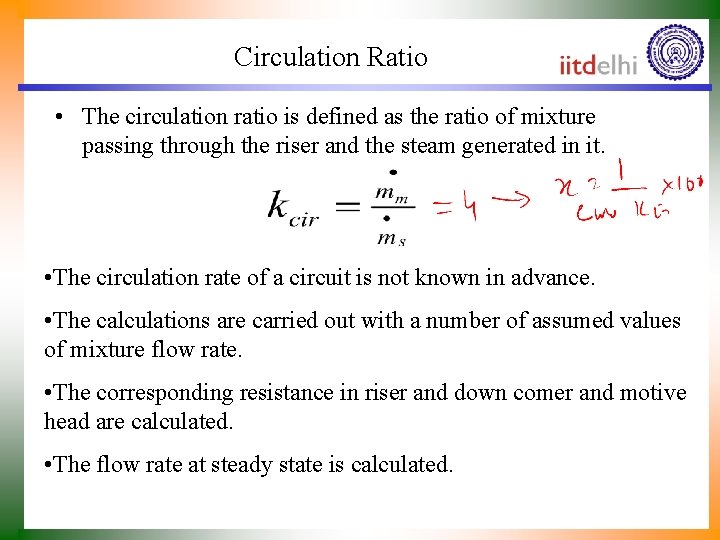
Circulation Ratio • The circulation ratio is defined as the ratio of mixture passing through the riser and the steam generated in it. • The circulation rate of a circuit is not known in advance. • The calculations are carried out with a number of assumed values of mixture flow rate. • The corresponding resistance in riser and down comer and motive head are calculated. • The flow rate at steady state is calculated.
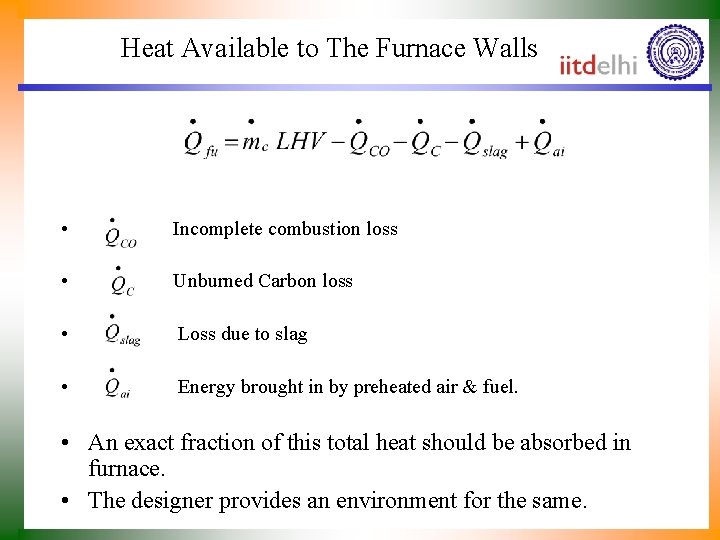
Heat Available to The Furnace Walls • Incomplete combustion loss • Unburned Carbon loss • Loss due to slag • Energy brought in by preheated air & fuel. • An exact fraction of this total heat should be absorbed in furnace. • The designer provides an environment for the same.
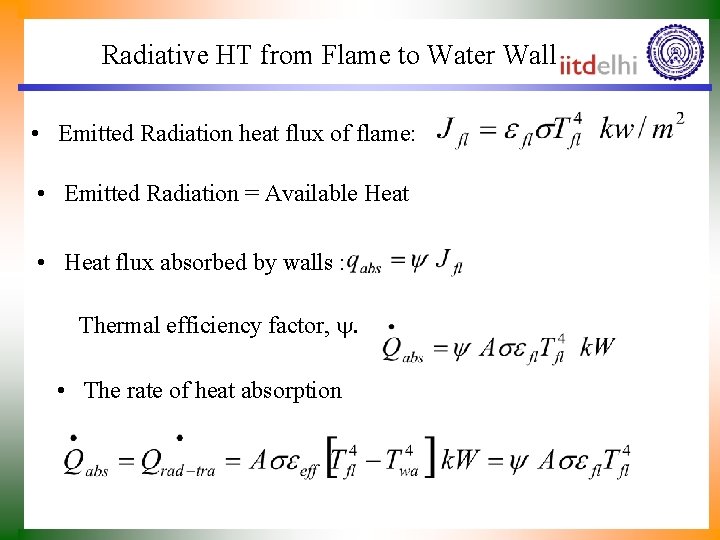
Radiative HT from Flame to Water Wall • Emitted Radiation heat flux of flame: • Emitted Radiation = Available Heat • Heat flux absorbed by walls : Thermal efficiency factor, y. • The rate of heat absorption
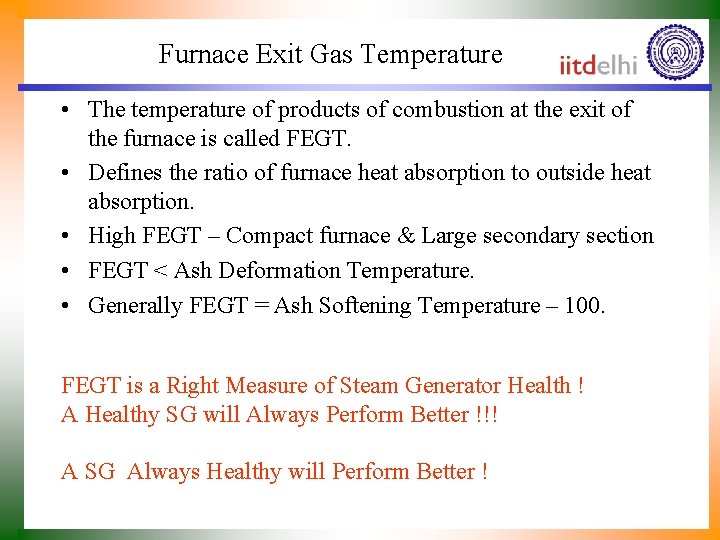
Furnace Exit Gas Temperature • The temperature of products of combustion at the exit of the furnace is called FEGT. • Defines the ratio of furnace heat absorption to outside heat absorption. • High FEGT – Compact furnace & Large secondary section • FEGT < Ash Deformation Temperature. • Generally FEGT = Ash Softening Temperature – 100. FEGT is a Right Measure of Steam Generator Health ! A Healthy SG will Always Perform Better !!! A SG Always Healthy will Perform Better !
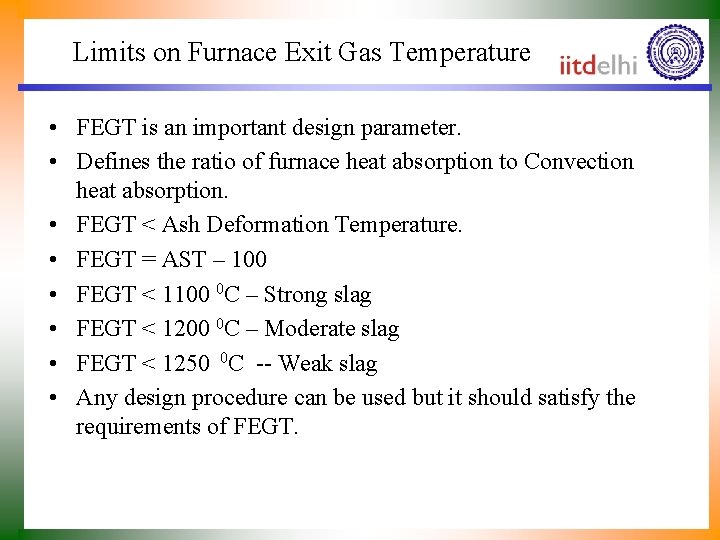
Limits on Furnace Exit Gas Temperature • FEGT is an important design parameter. • Defines the ratio of furnace heat absorption to Convection heat absorption. • FEGT < Ash Deformation Temperature. • FEGT = AST – 100 • FEGT < 1100 0 C – Strong slag • FEGT < 1200 0 C – Moderate slag • FEGT < 1250 0 C -- Weak slag • Any design procedure can be used but it should satisfy the requirements of FEGT.
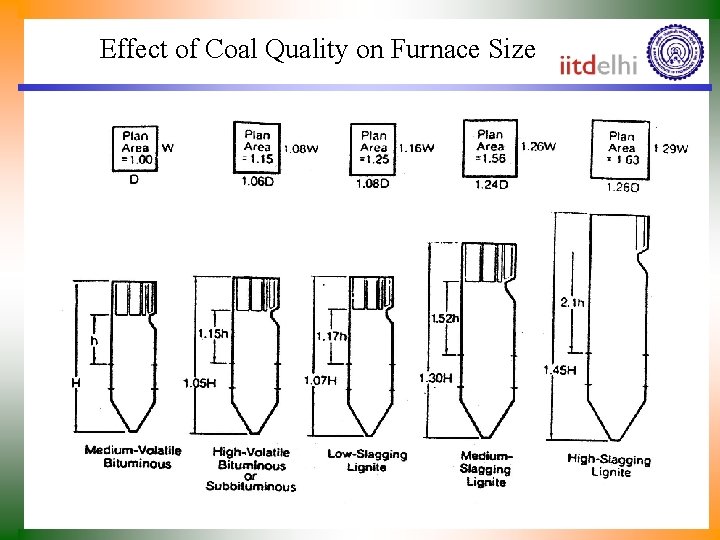
Effect of Coal Quality on Furnace Size
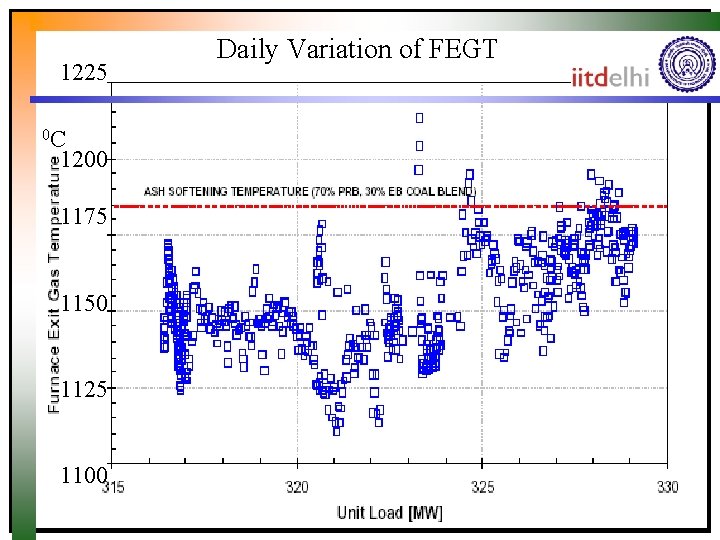
1225 0 C 1200 1175 1150 1125 1100 Daily Variation of FEGT
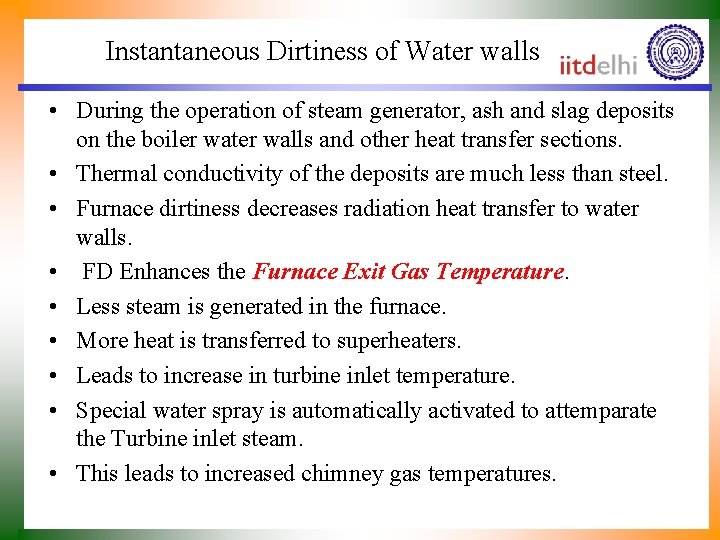
Instantaneous Dirtiness of Water walls • During the operation of steam generator, ash and slag deposits on the boiler water walls and other heat transfer sections. • Thermal conductivity of the deposits are much less than steel. • Furnace dirtiness decreases radiation heat transfer to water walls. • FD Enhances the Furnace Exit Gas Temperature. • Less steam is generated in the furnace. • More heat is transferred to superheaters. • Leads to increase in turbine inlet temperature. • Special water spray is automatically activated to attemparate the Turbine inlet steam. • This leads to increased chimney gas temperatures.
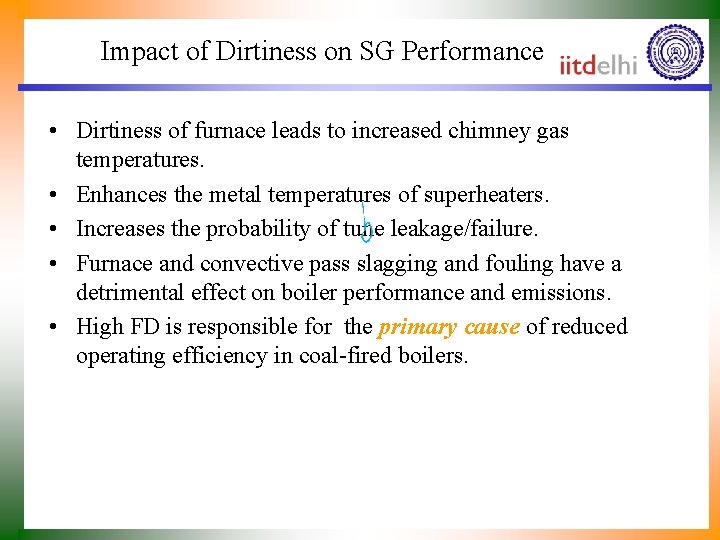
Impact of Dirtiness on SG Performance • Dirtiness of furnace leads to increased chimney gas temperatures. • Enhances the metal temperatures of superheaters. • Increases the probability of tune leakage/failure. • Furnace and convective pass slagging and fouling have a detrimental effect on boiler performance and emissions. • High FD is responsible for the primary cause of reduced operating efficiency in coal-fired boilers.
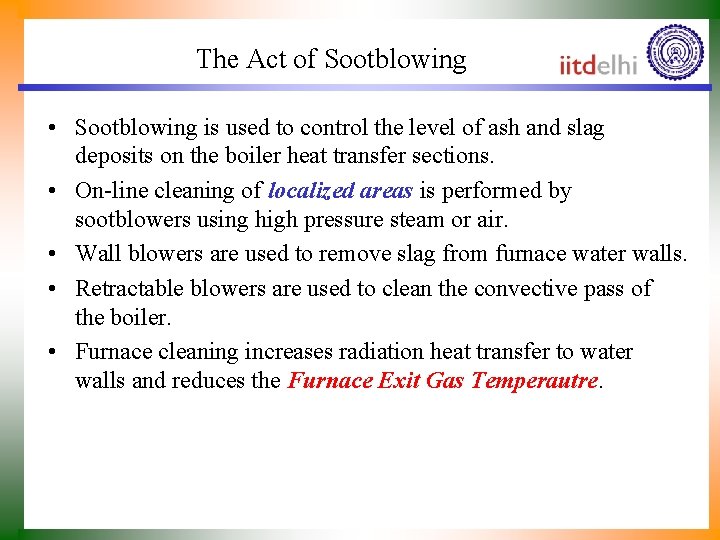
The Act of Sootblowing • Sootblowing is used to control the level of ash and slag deposits on the boiler heat transfer sections. • On-line cleaning of localized areas is performed by sootblowers using high pressure steam or air. • Wall blowers are used to remove slag from furnace water walls. • Retractable blowers are used to clean the convective pass of the boiler. • Furnace cleaning increases radiation heat transfer to water walls and reduces the Furnace Exit Gas Temperautre.
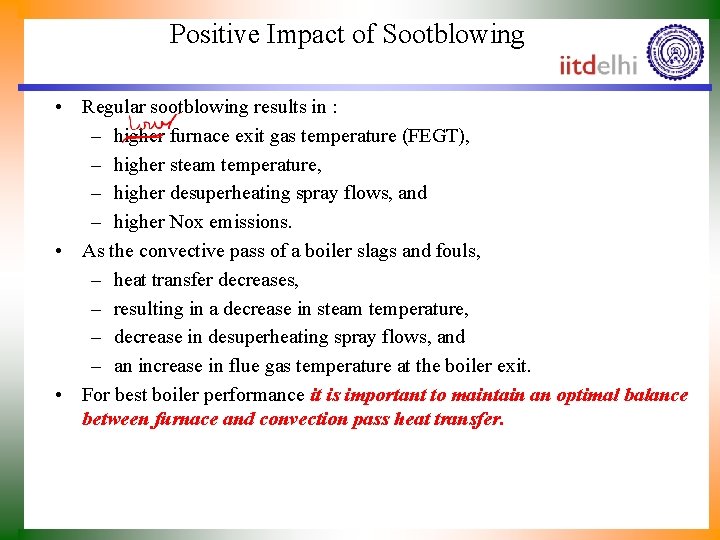
Positive Impact of Sootblowing • Regular sootblowing results in : – higher furnace exit gas temperature (FEGT), – higher steam temperature, – higher desuperheating spray flows, and – higher Nox emissions. • As the convective pass of a boiler slags and fouls, – heat transfer decreases, – resulting in a decrease in steam temperature, – decrease in desuperheating spray flows, and – an increase in flue gas temperature at the boiler exit. • For best boiler performance it is important to maintain an optimal balance between furnace and convection pass heat transfer.
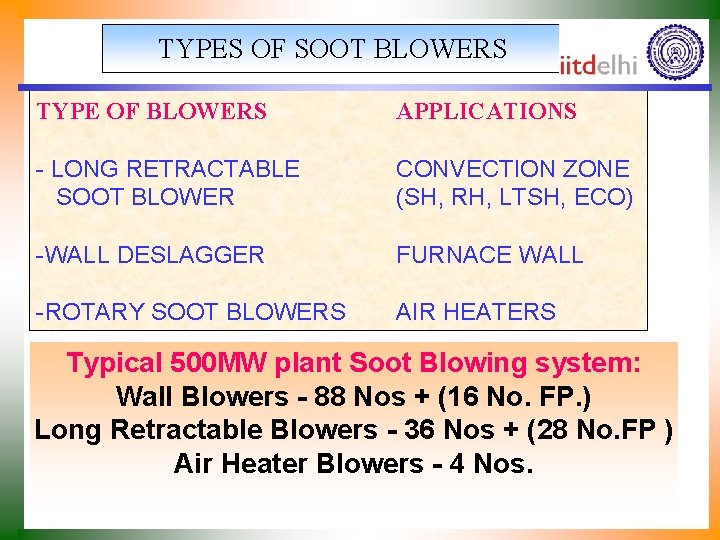
TYPES OF SOOT BLOWERS TYPE OF BLOWERS APPLICATIONS - LONG RETRACTABLE SOOT BLOWER CONVECTION ZONE (SH, RH, LTSH, ECO) -WALL DESLAGGER FURNACE WALL -ROTARY SOOT BLOWERS AIR HEATERS Typical 500 MW plant Soot Blowing system: Wall Blowers - 88 Nos + (16 No. FP. ) Long Retractable Blowers - 36 Nos + (28 No. FP ) Air Heater Blowers - 4 Nos.
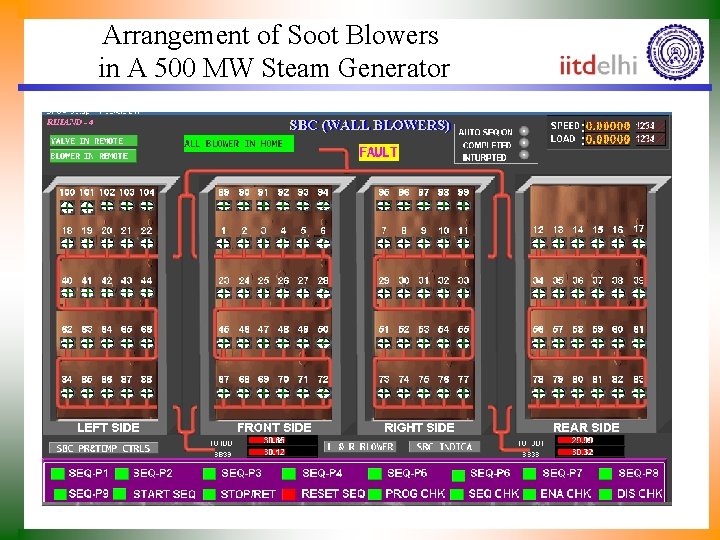
Arrangement of Soot Blowers in A 500 MW Steam Generator
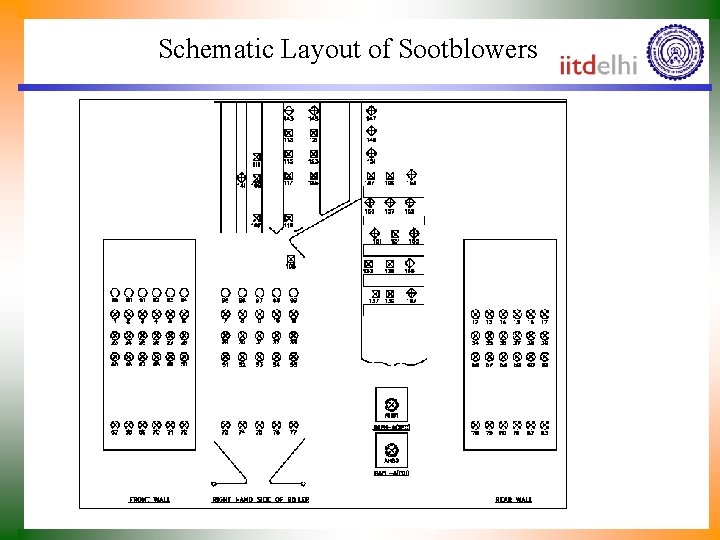
Schematic Layout of Sootblowers
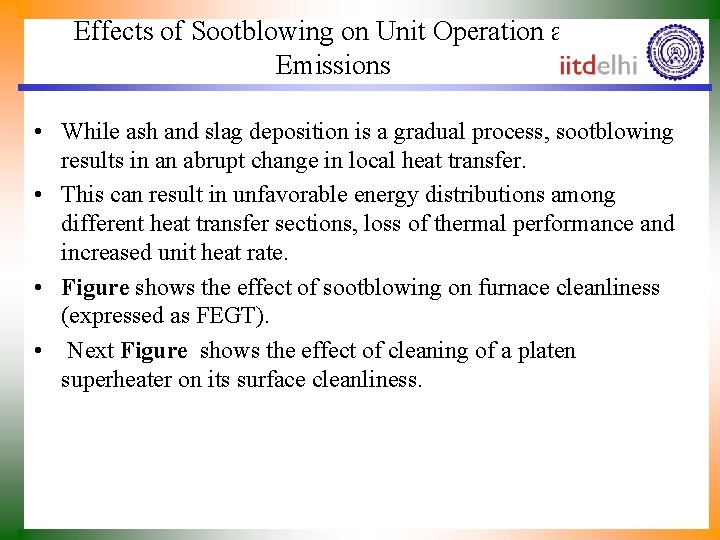
Effects of Sootblowing on Unit Operation and Emissions • While ash and slag deposition is a gradual process, sootblowing results in an abrupt change in local heat transfer. • This can result in unfavorable energy distributions among different heat transfer sections, loss of thermal performance and increased unit heat rate. • Figure shows the effect of sootblowing on furnace cleanliness (expressed as FEGT). • Next Figure shows the effect of cleaning of a platen superheater on its surface cleanliness.
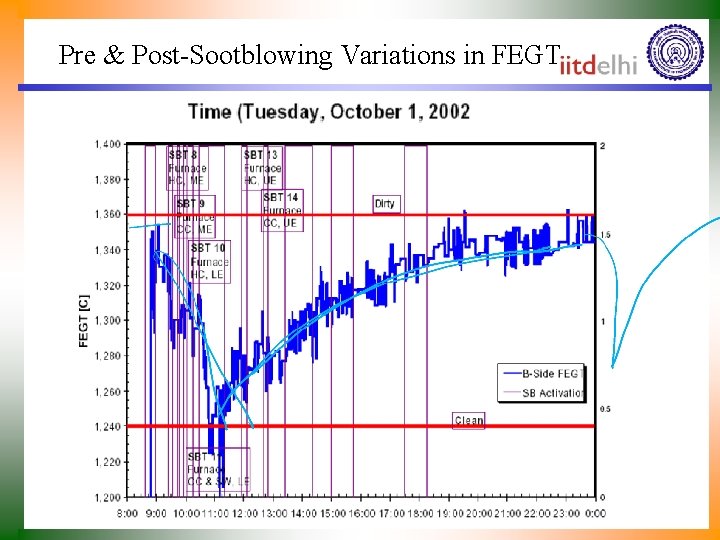
Pre & Post-Sootblowing Variations in FEGT
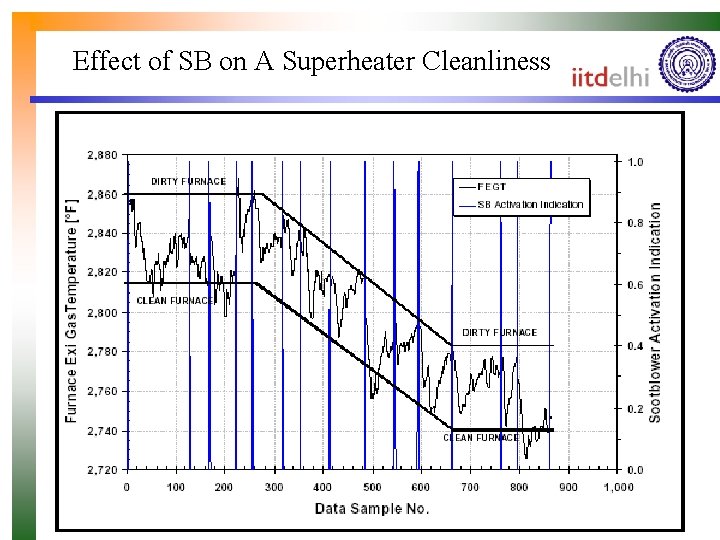
Effect of SB on A Superheater Cleanliness
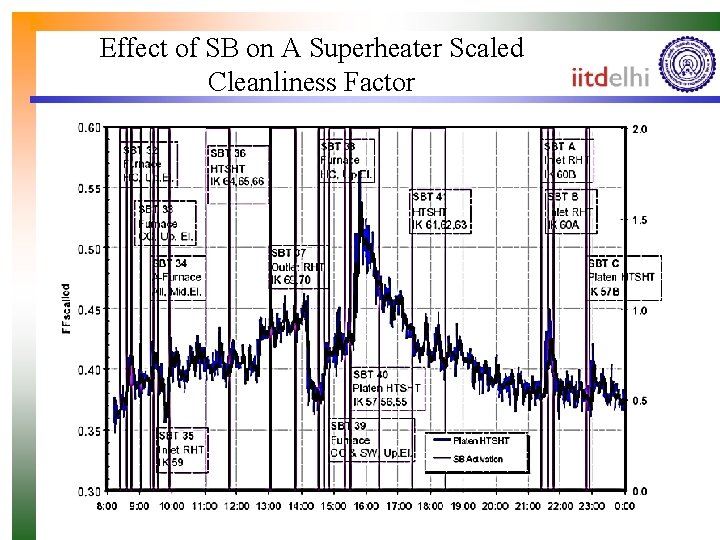
Effect of SB on A Superheater Scaled Cleanliness Factor
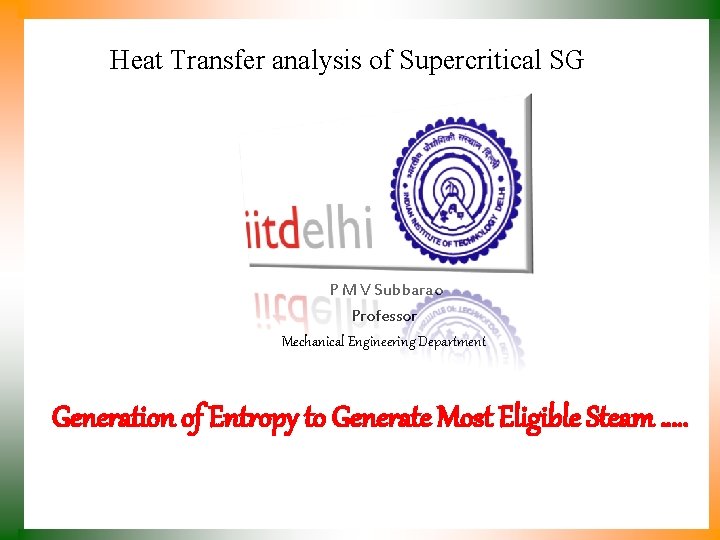
Heat Transfer analysis of Supercritical SG P M V Subbarao Professor Mechanical Engineering Department Generation of Entropy to Generate Most Eligible Steam …. .
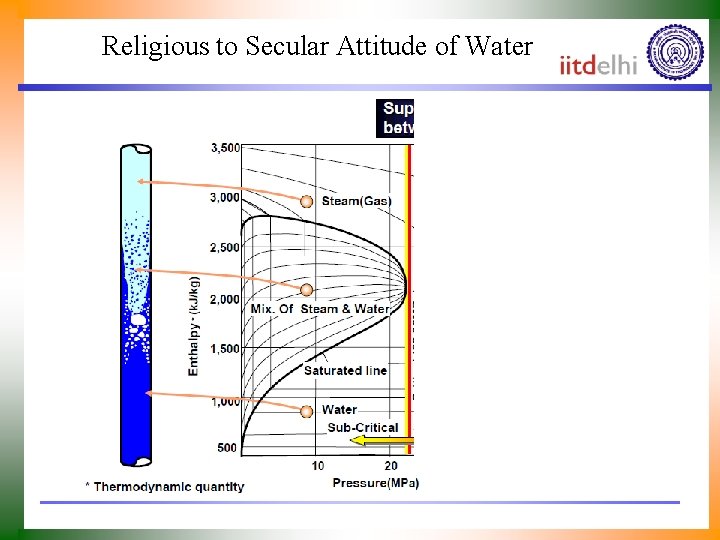
Religious to Secular Attitude of Water
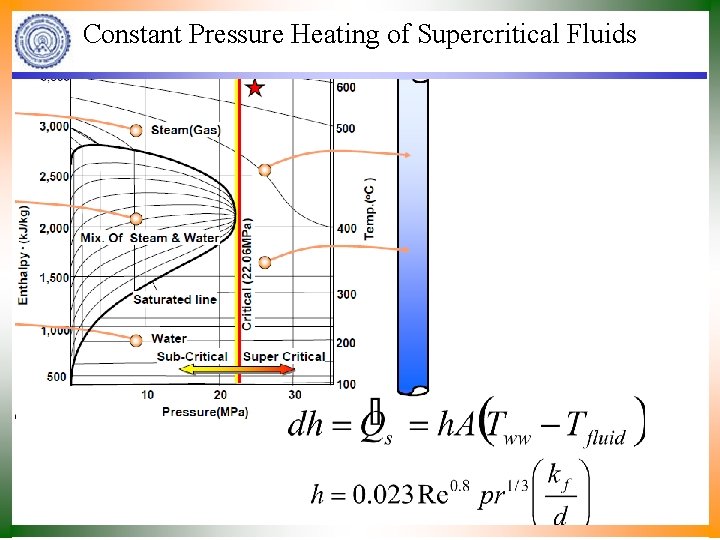
Constant Pressure Heating of Supercritical Fluids
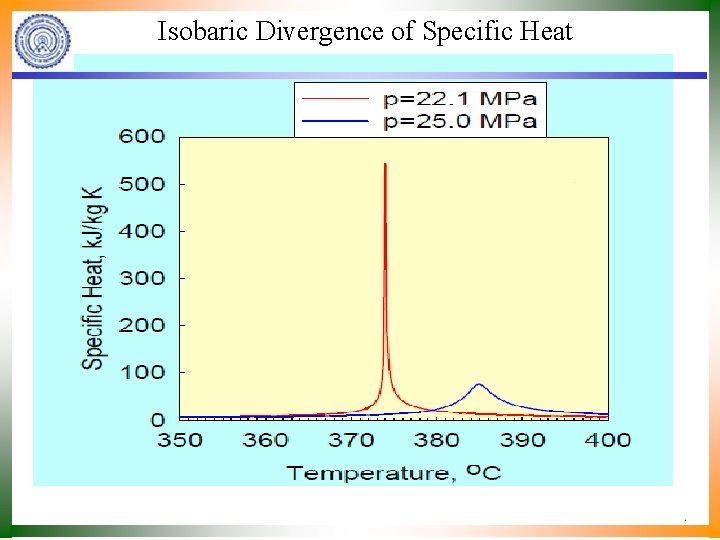
Isobaric Divergence of Specific Heat
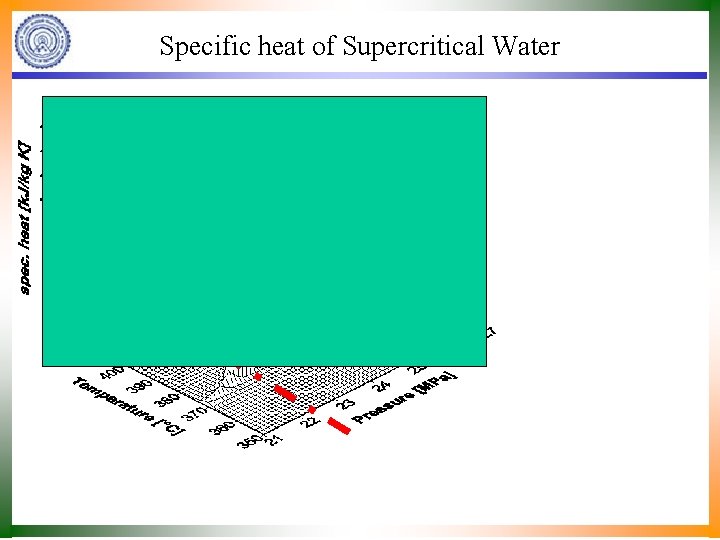
Specific heat of Supercritical Water
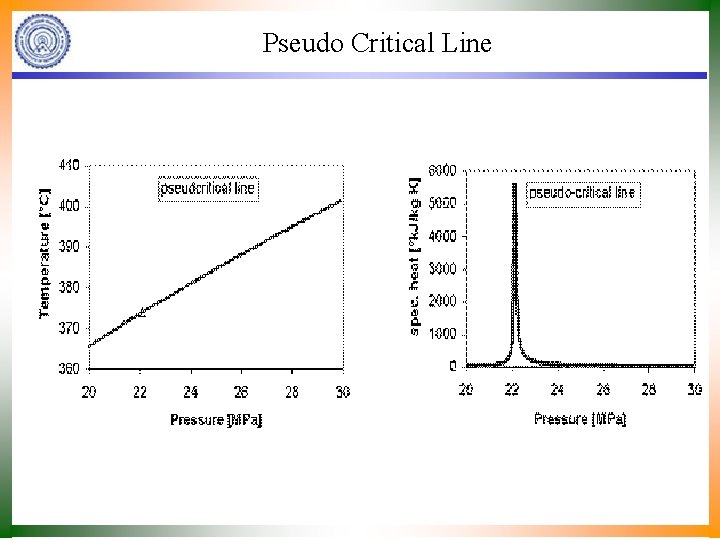
Pseudo Critical Line
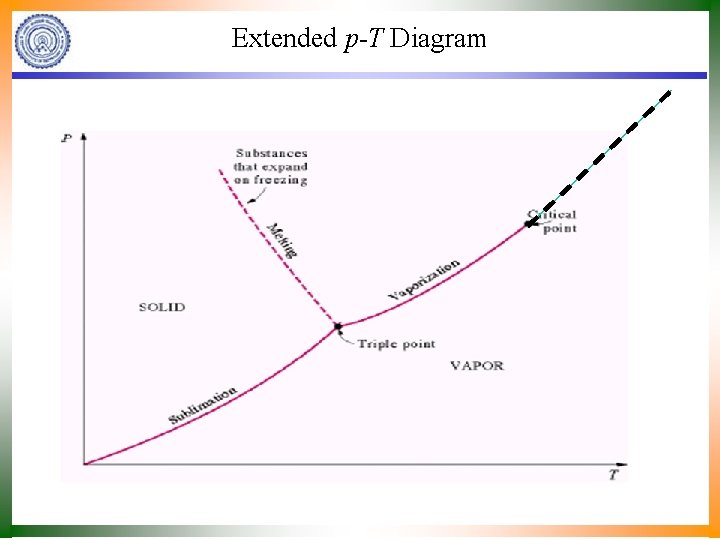
Extended p-T Diagram
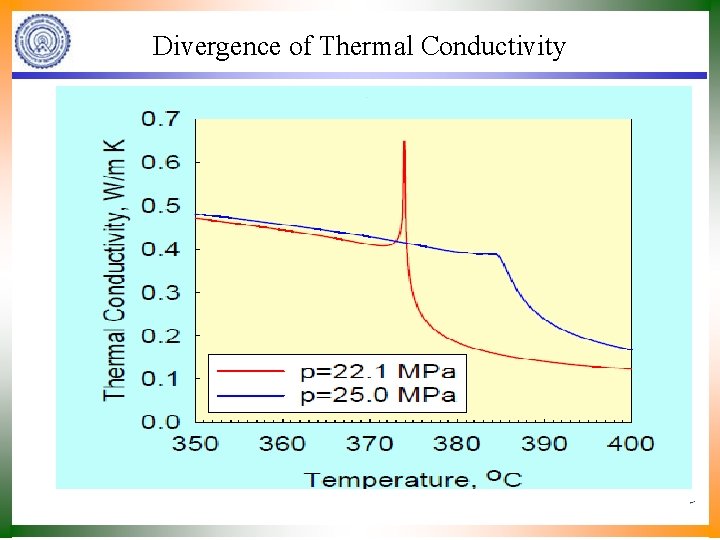
Divergence of Thermal Conductivity
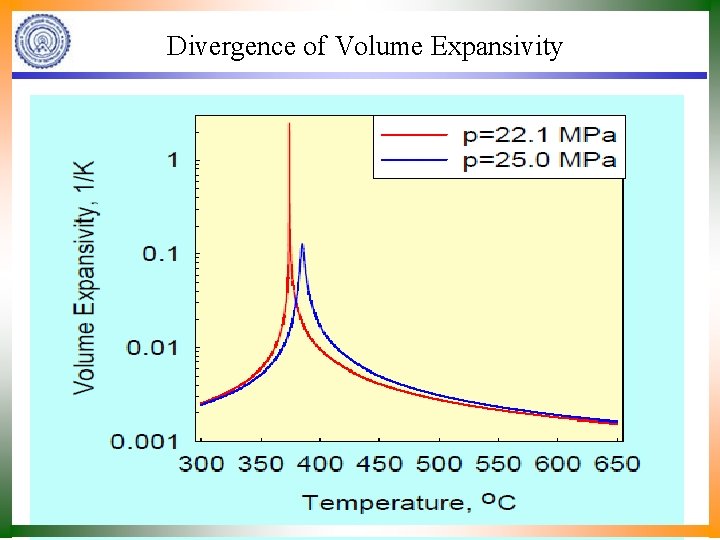
Divergence of Volume Expansivity
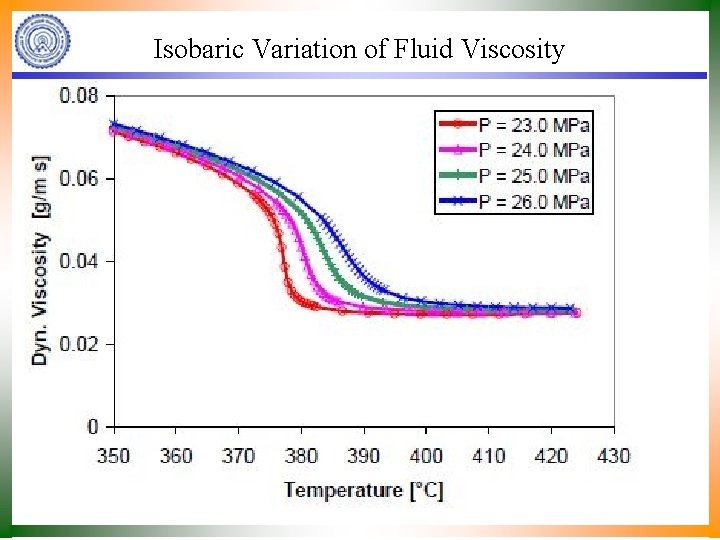
Isobaric Variation of Fluid Viscosity
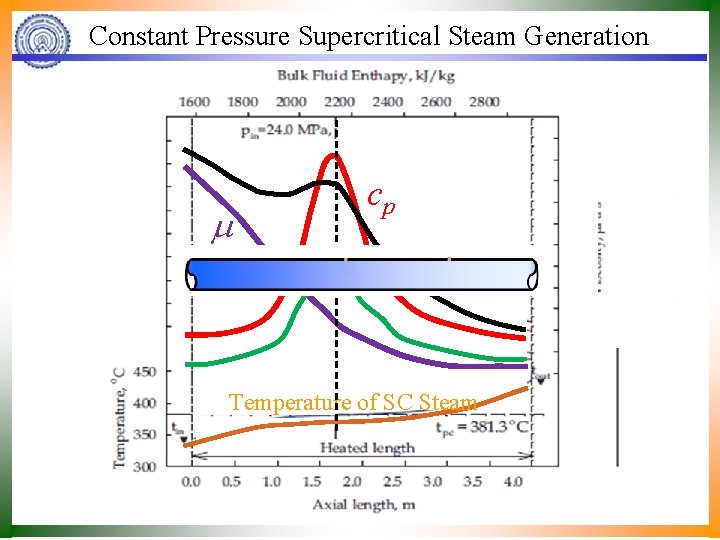
Constant Pressure Supercritical Steam Generation cp Pr k Temperature of SC Steam
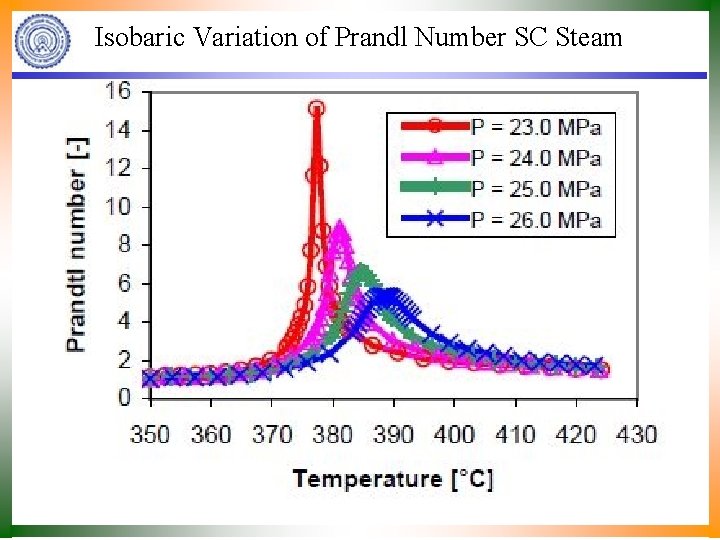
Isobaric Variation of Prandl Number SC Steam
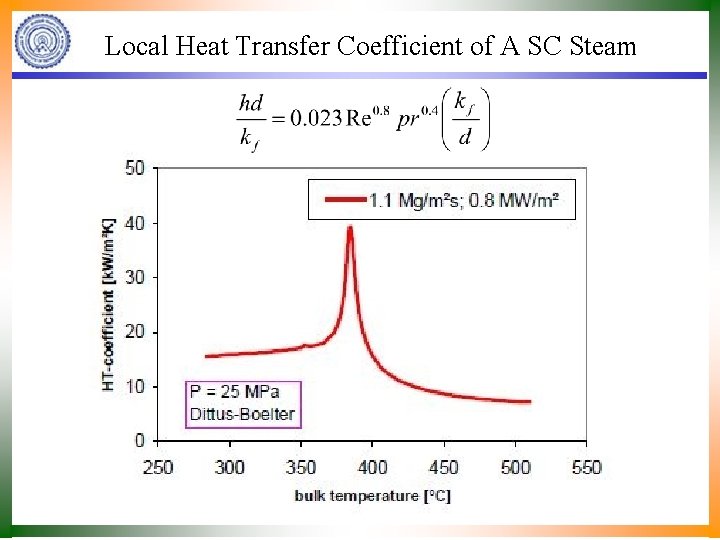
Local Heat Transfer Coefficient of A SC Steam
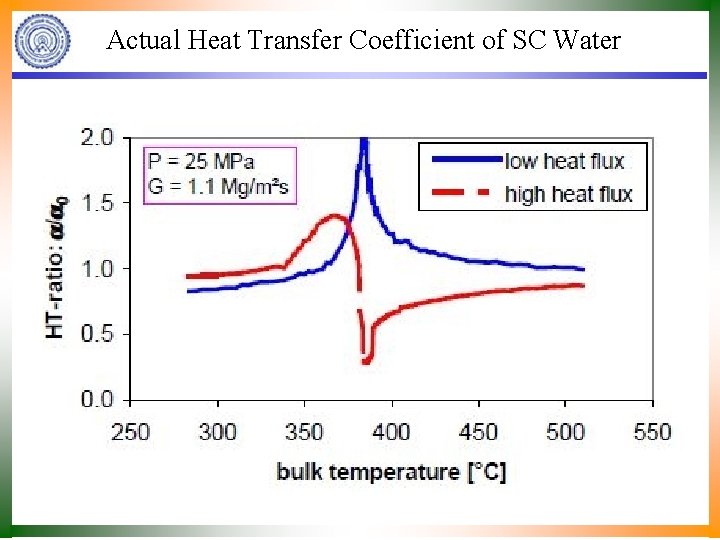
Actual Heat Transfer Coefficient of SC Water
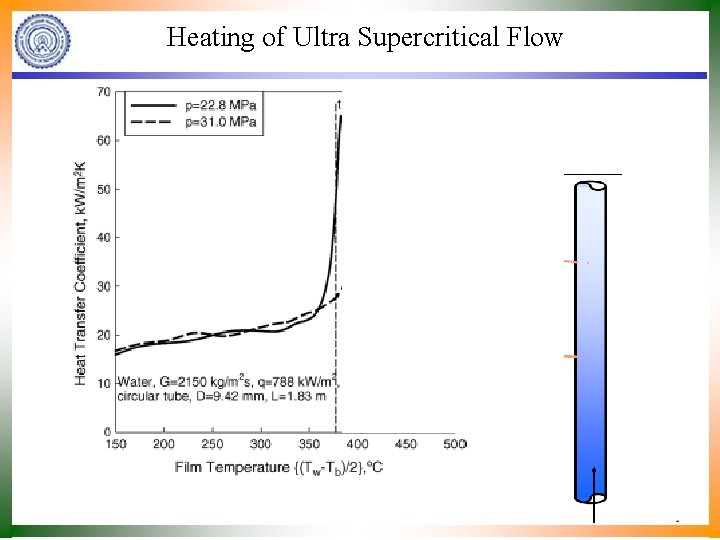
Heating of Ultra Supercritical Flow
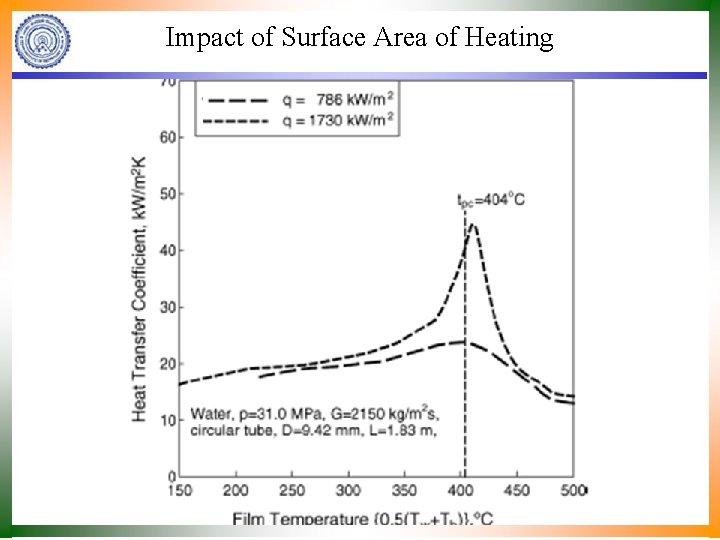
Impact of Surface Area of Heating
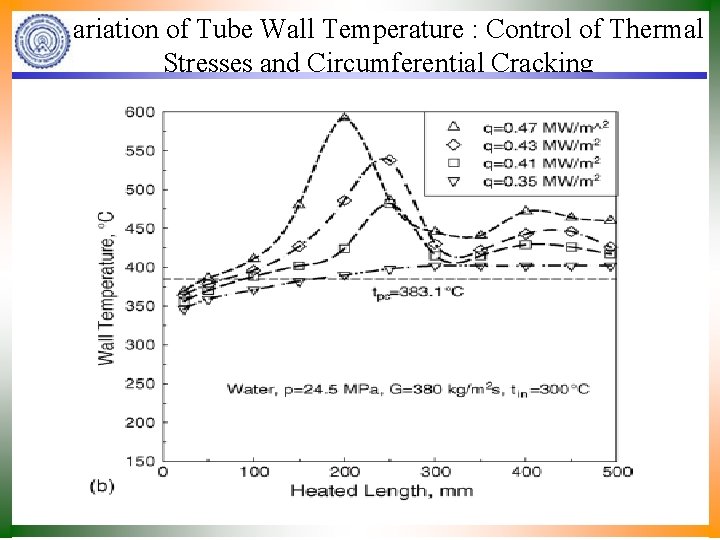
Variation of Tube Wall Temperature : Control of Thermal Stresses and Circumferential Cracking
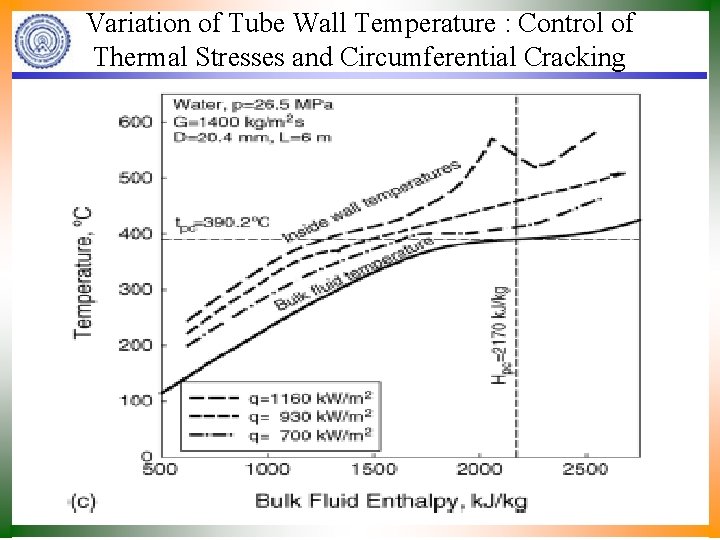
Variation of Tube Wall Temperature : Control of Thermal Stresses and Circumferential Cracking
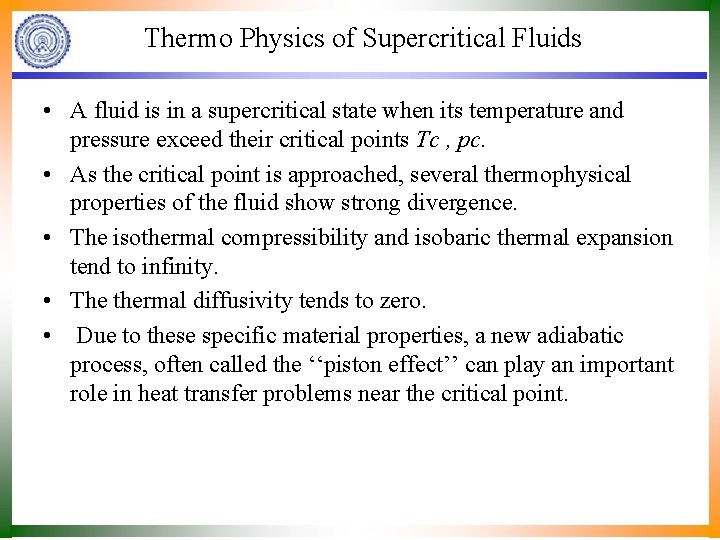
Thermo Physics of Supercritical Fluids • A fluid is in a supercritical state when its temperature and pressure exceed their critical points Tc , pc. • As the critical point is approached, several thermophysical properties of the fluid show strong divergence. • The isothermal compressibility and isobaric thermal expansion tend to infinity. • The thermal diffusivity tends to zero. • Due to these specific material properties, a new adiabatic process, often called the ‘‘piston effect’’ can play an important role in heat transfer problems near the critical point.
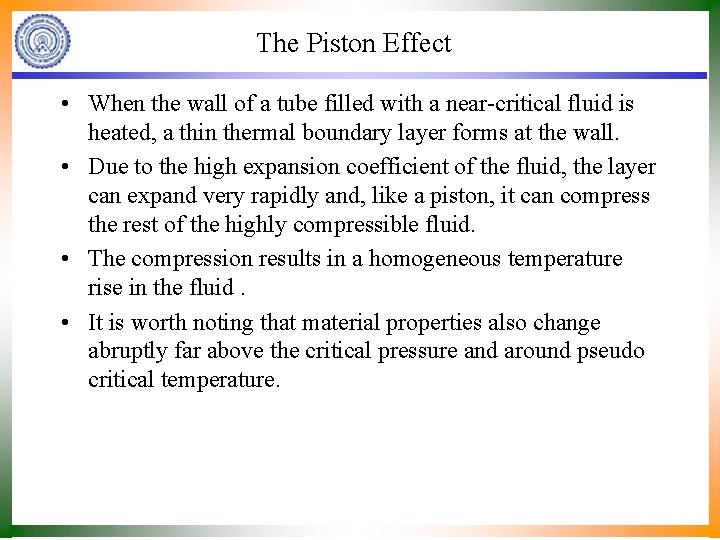
The Piston Effect • When the wall of a tube filled with a near-critical fluid is heated, a thin thermal boundary layer forms at the wall. • Due to the high expansion coefficient of the fluid, the layer can expand very rapidly and, like a piston, it can compress the rest of the highly compressible fluid. • The compression results in a homogeneous temperature rise in the fluid. • It is worth noting that material properties also change abruptly far above the critical pressure and around pseudo critical temperature.
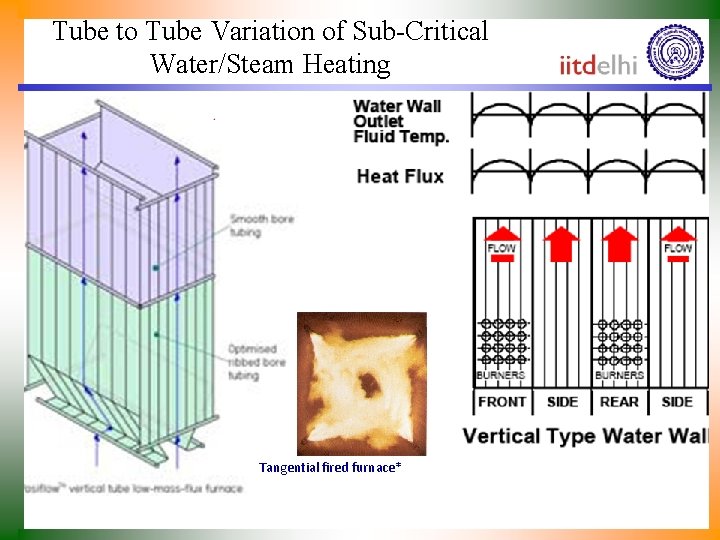
Tube to Tube Variation of Sub-Critical Water/Steam Heating Tangential fired furnace*
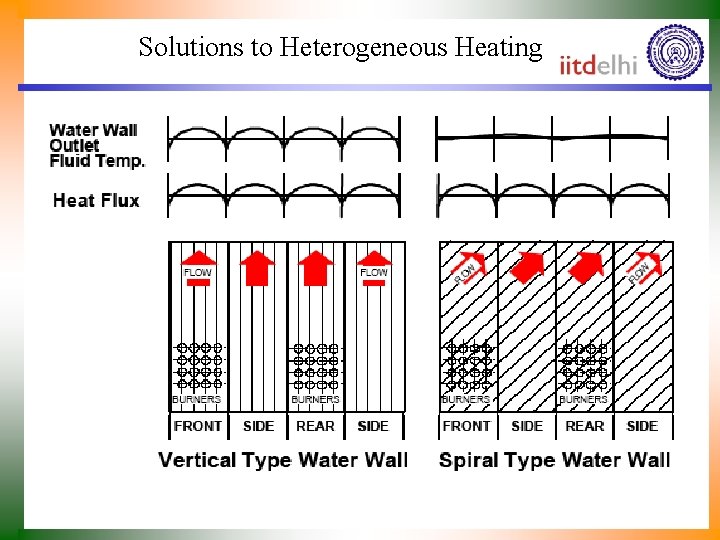
Solutions to Heterogeneous Heating
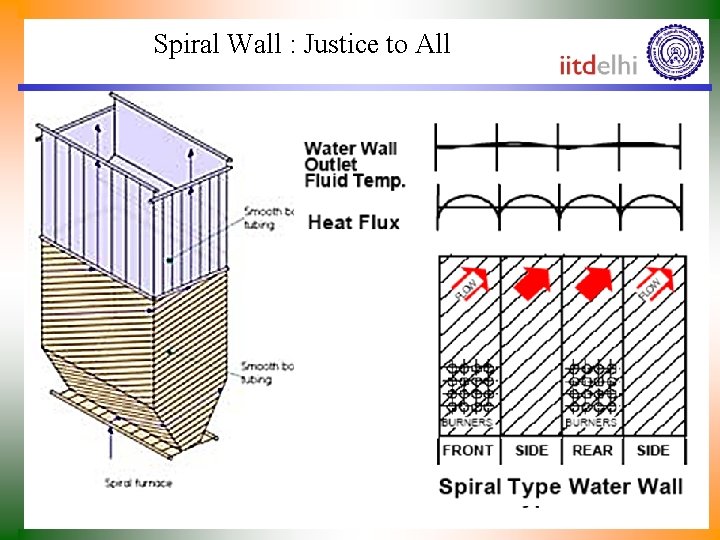
Spiral Wall : Justice to All
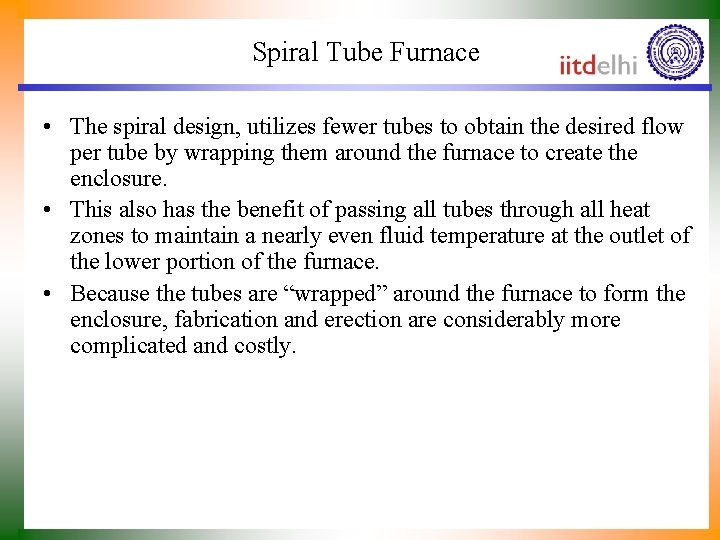
Spiral Tube Furnace • The spiral design, utilizes fewer tubes to obtain the desired flow per tube by wrapping them around the furnace to create the enclosure. • This also has the benefit of passing all tubes through all heat zones to maintain a nearly even fluid temperature at the outlet of the lower portion of the furnace. • Because the tubes are “wrapped” around the furnace to form the enclosure, fabrication and erection are considerably more complicated and costly.
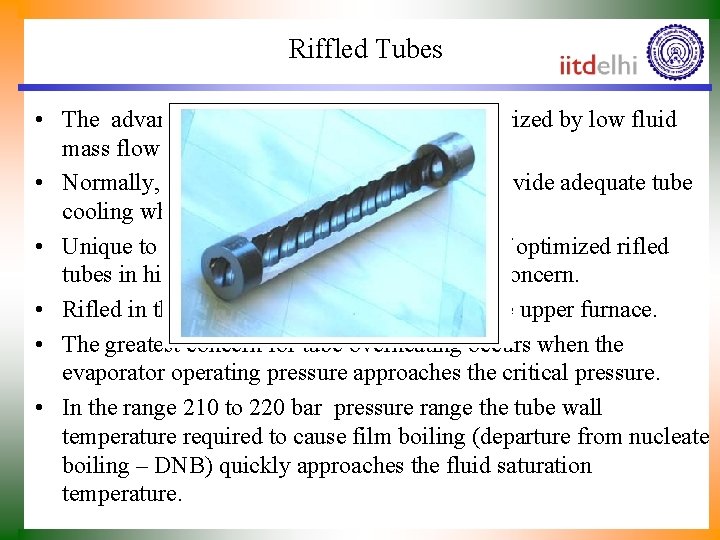
Riffled Tubes • The advanced Vertical technology is characterized by low fluid mass flow rates. • Normally, low fluid mass flow rates do not provide adequate tube cooling when used with smooth tubing. • Unique to the Vertical technology is the use of optimized rifled tubes in high heat flux areas to eliminate this concern. • Rifled in the lower furnace, smooth-bore in the upper furnace. • The greatest concern for tube overheating occurs when the evaporator operating pressure approaches the critical pressure. • In the range 210 to 220 bar pressure range the tube wall temperature required to cause film boiling (departure from nucleate boiling – DNB) quickly approaches the fluid saturation temperature.
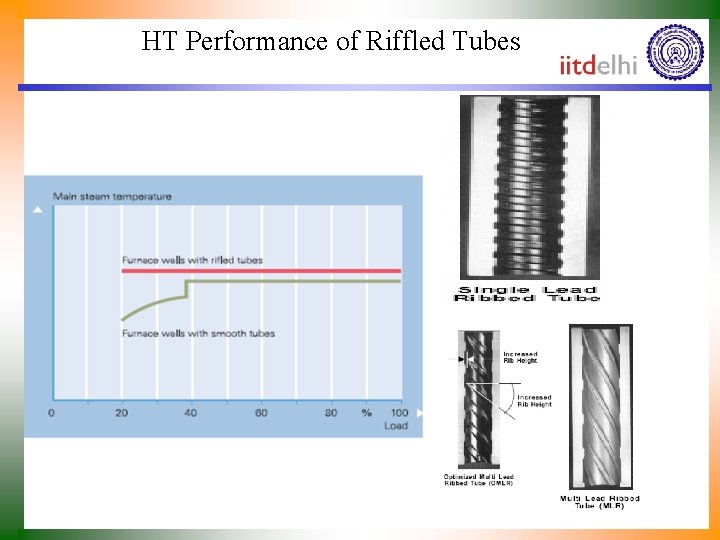
HT Performance of Riffled Tubes
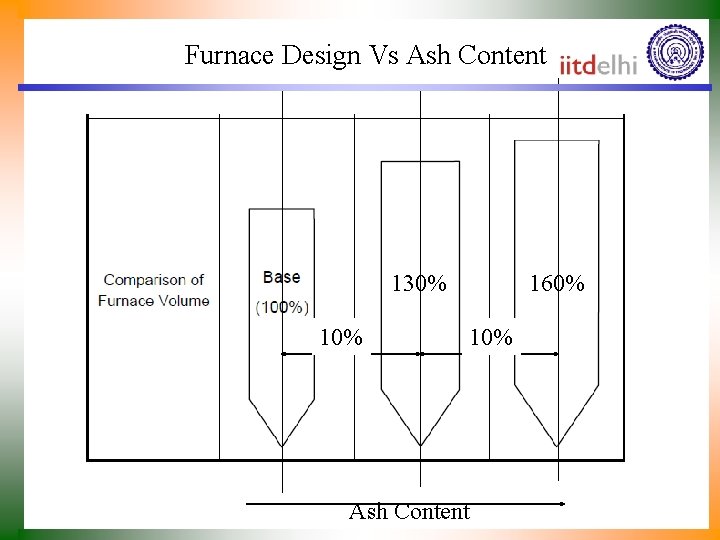
Furnace Design Vs Ash Content 130% 160% 10% Ash Content
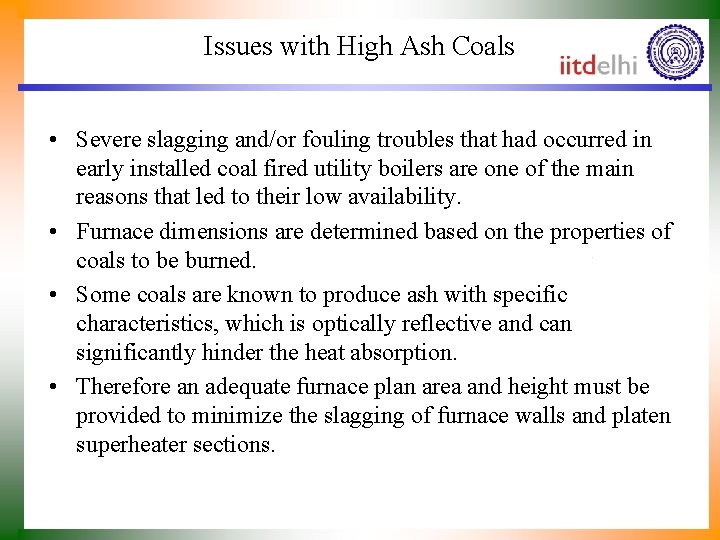
Issues with High Ash Coals • Severe slagging and/or fouling troubles that had occurred in early installed coal fired utility boilers are one of the main reasons that led to their low availability. • Furnace dimensions are determined based on the properties of coals to be burned. • Some coals are known to produce ash with specific characteristics, which is optically reflective and can significantly hinder the heat absorption. • Therefore an adequate furnace plan area and height must be provided to minimize the slagging of furnace walls and platen superheater sections.
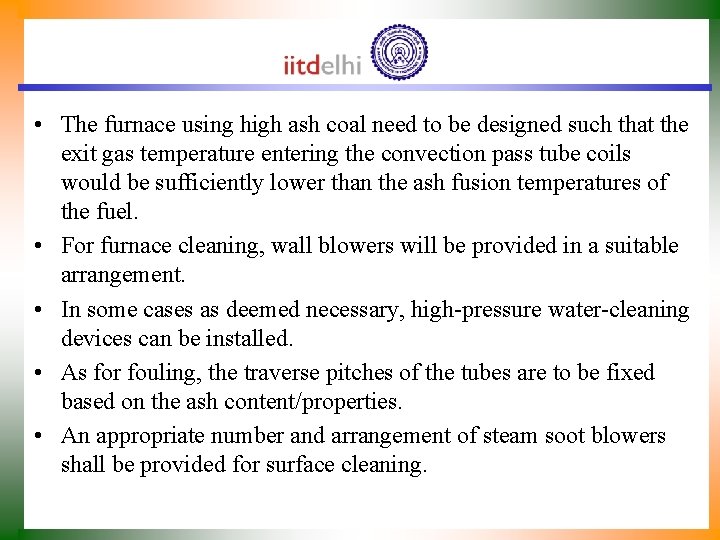
• The furnace using high ash coal need to be designed such that the exit gas temperature entering the convection pass tube coils would be sufficiently lower than the ash fusion temperatures of the fuel. • For furnace cleaning, wall blowers will be provided in a suitable arrangement. • In some cases as deemed necessary, high-pressure water-cleaning devices can be installed. • As for fouling, the traverse pitches of the tubes are to be fixed based on the ash content/properties. • An appropriate number and arrangement of steam soot blowers shall be provided for surface cleaning.
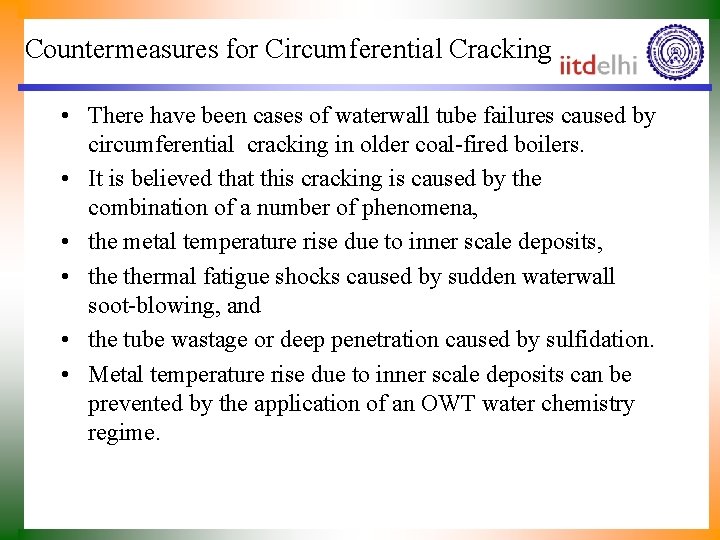
Countermeasures for Circumferential Cracking • There have been cases of waterwall tube failures caused by circumferential cracking in older coal-fired boilers. • It is believed that this cracking is caused by the combination of a number of phenomena, • the metal temperature rise due to inner scale deposits, • thermal fatigue shocks caused by sudden waterwall soot-blowing, and • the tube wastage or deep penetration caused by sulfidation. • Metal temperature rise due to inner scale deposits can be prevented by the application of an OWT water chemistry regime.
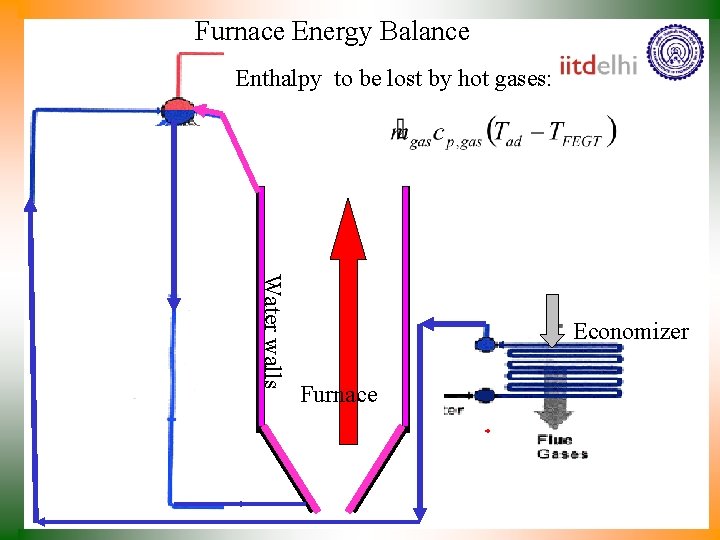
Furnace Energy Balance Enthalpy to be lost by hot gases: Water walls Economizer Furnace
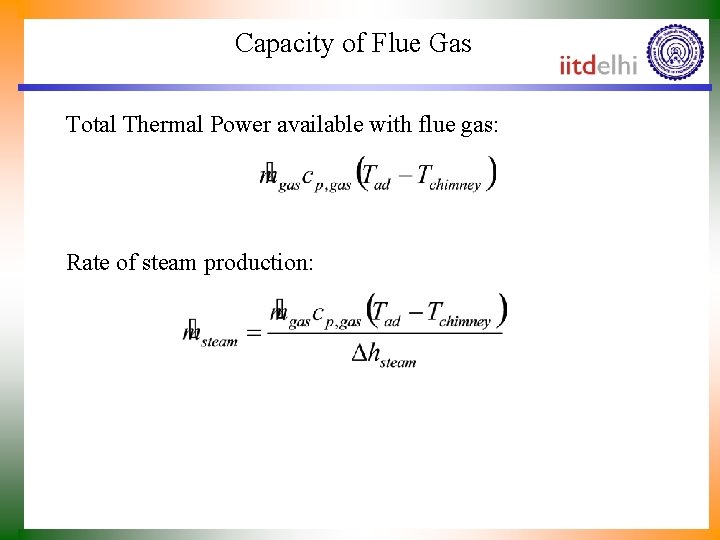
Capacity of Flue Gas Total Thermal Power available with flue gas: Rate of steam production:
Dittus boelter equation
Steam steam generator erosion
Steam steam generator fouling
Steam generation theory
Steam boiler heat exchanger
Steam condenser heat exchanger
Ntu method
Single pass heat exchanger
Me 421
First generation antipsychotics
We worship you hallelujah
Heat generation
Naumann reversion reaction
Electric arc furnace
Disadvantages of induction furnace
Induction tunnel furnace
Who shot john wilkes booth
Exothermic atmosphere furnace
Daniel's friends in the fiery furnace
Fearthine
Furnace
How is comparative analysis used in engineering economy?
Daniel's friends in the fiery furnace
Rotary hearth furnace
Blast furnace charge
Introductory performance review
Ams2750 furnace class
Furnace solenoid
Furnace atmosphere control
Sealed quench furnace wikipedia
Asme 2750 furnaces
Fortis furnace commissioning sheet
Chapter 21 the furnace of civil war
Electric arc furnace temperature
Flame rectification
A disturbance that transfers energy is called
Heat transfer coefficient table
Heat transfer cengel
The overall heat transfer coefficient
Popcorn heat transfer worksheet
Convection definition
Advection heat transfer
Governing equation heat transfer
Studyjams heat
How does heat move
Rubber is a good insulator of blank
Insulators at home
Radial heat conduction
Heat transfer objectives
Transient conduction means
Introduction to convection
Yunus cengel
Kanolu
Extended surface heat transfer experiment
Transfer function method cooling load
Examples of radiation
Critical radius of insulation for cylinder
Popcorn heat transfer lab answer key
Wisc online heat transfer
The ability to do work or transfer heat
Cylinder heat transfer
Electrical analogy in heat transfer
Conduction or convection
Bioprocess mass transfer
Rapid heat transfer
Heat and mass transfer
Conservation of energy heat transfer
Q heat energy
Chapter 7 heat transfer and change of phase
Linear heat conduction lab report
Radiation heat transfer examples
Heat transfer final
Example of heat transfer by radiation
Example of conduction
The overall heat transfer coefficient
View factor heat transfer
Heat transfer subject code
Heat transfer in polytropic process