ENT 249 ELECTRICAL CIRCUIT AND MACHINE Rotating Electrical
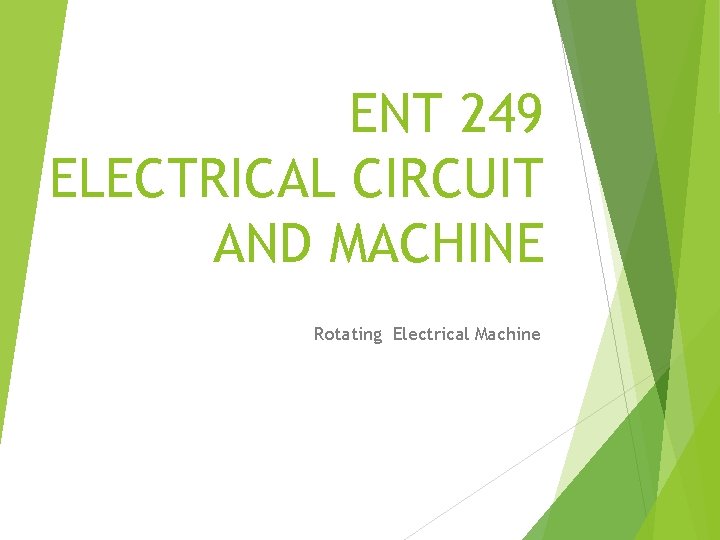
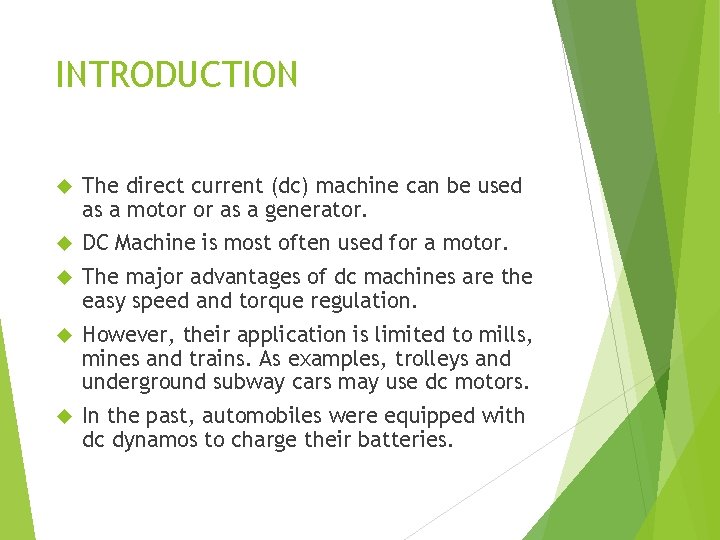
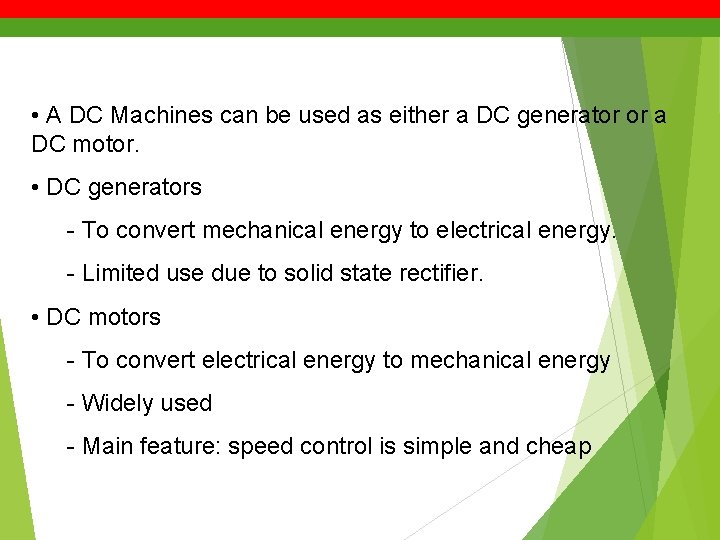
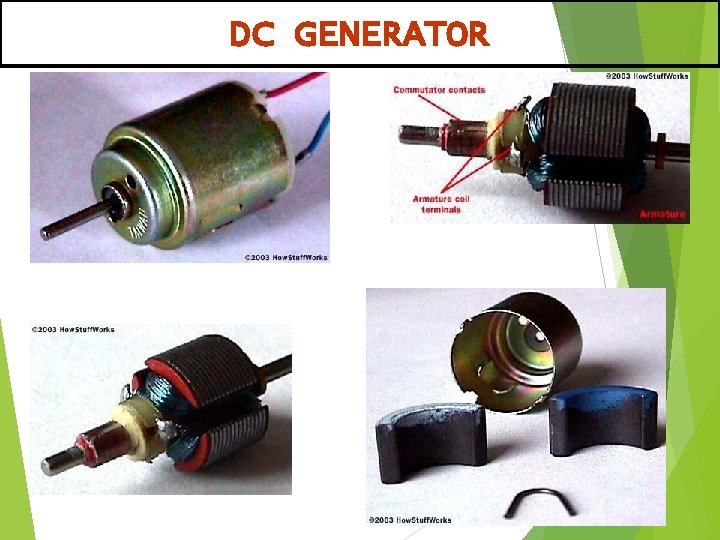
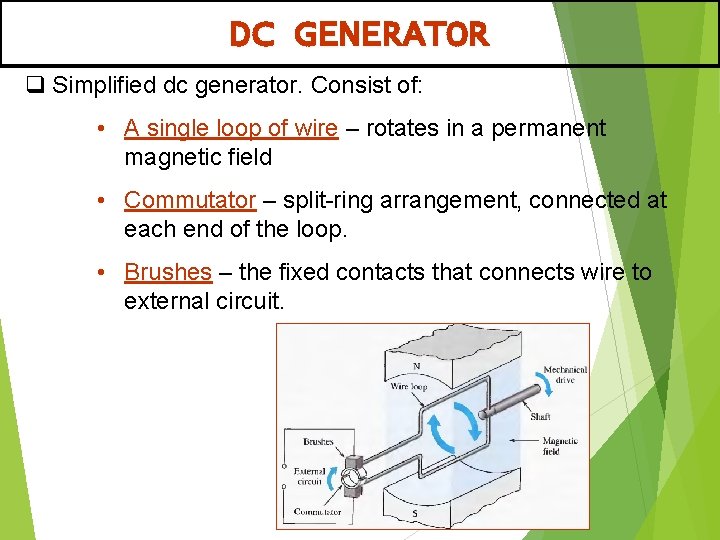
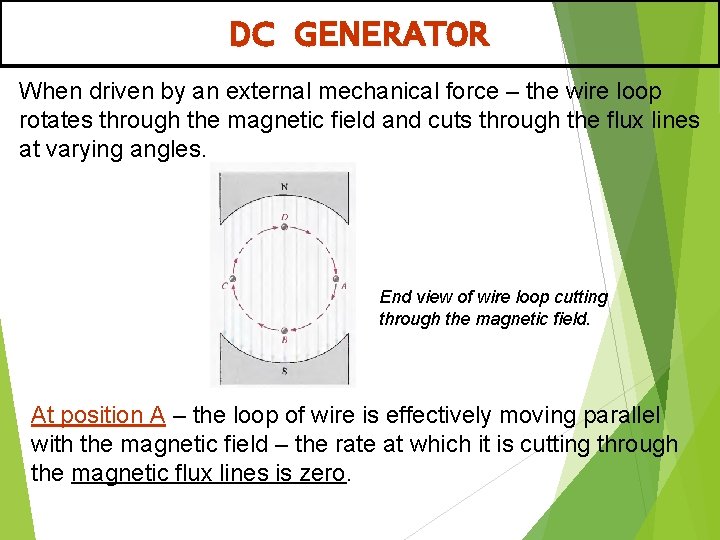
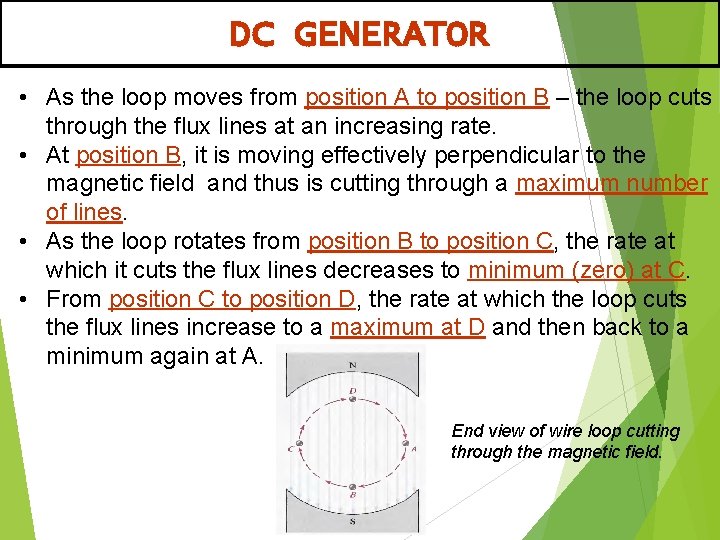
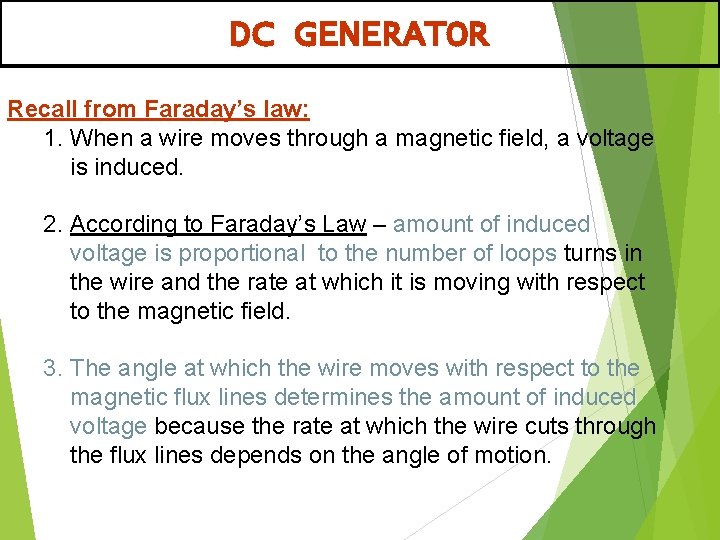
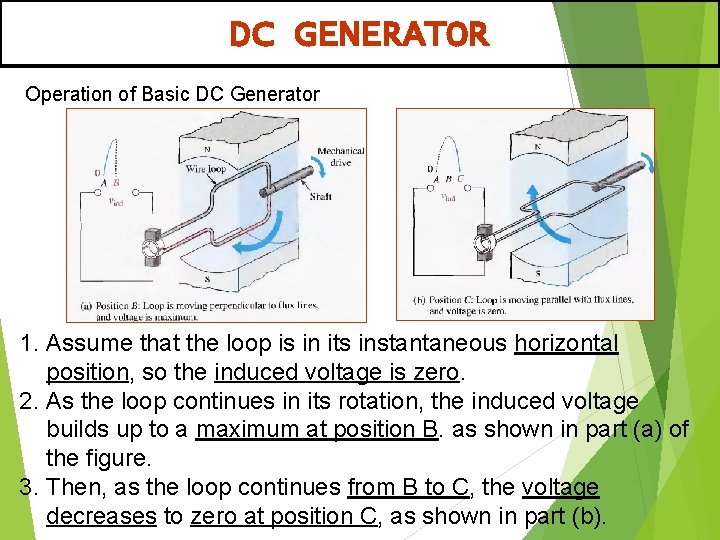
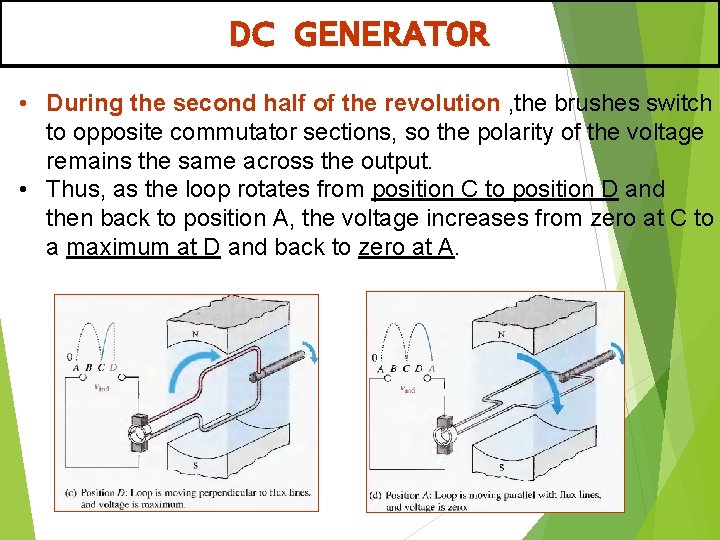
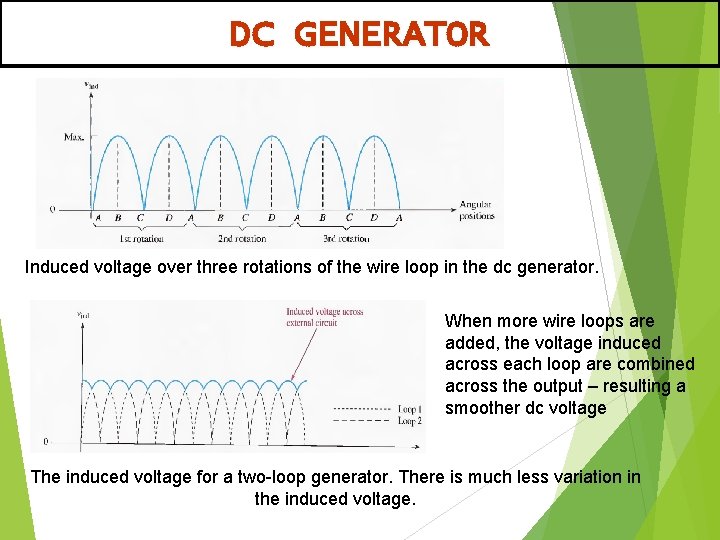
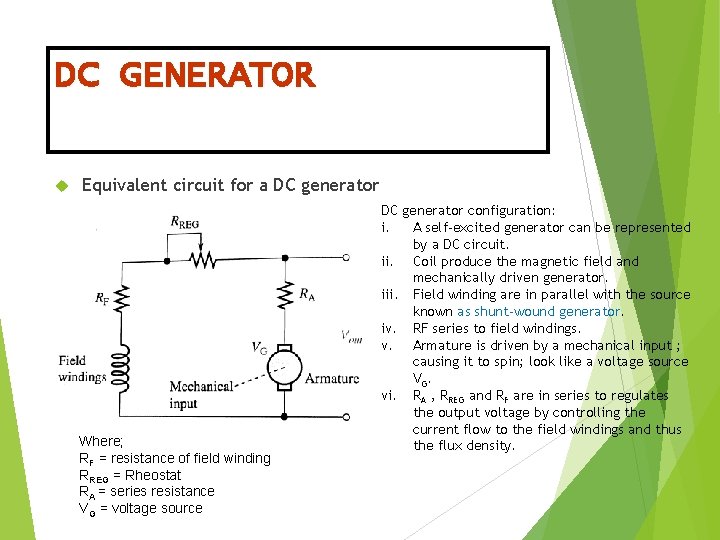
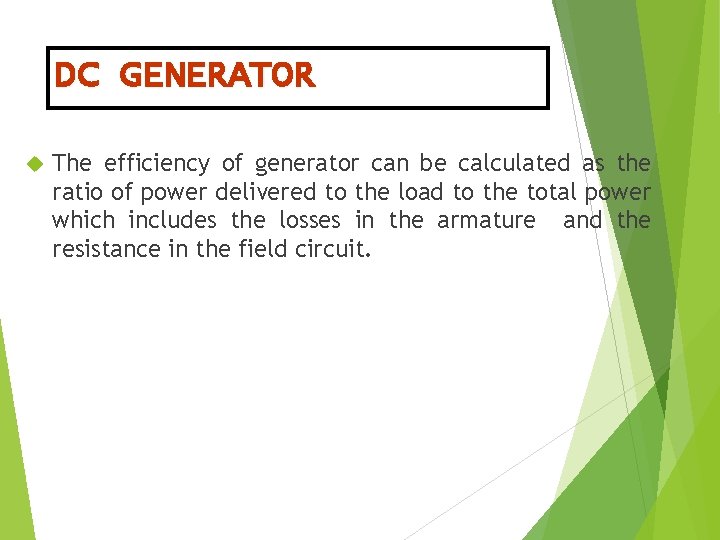
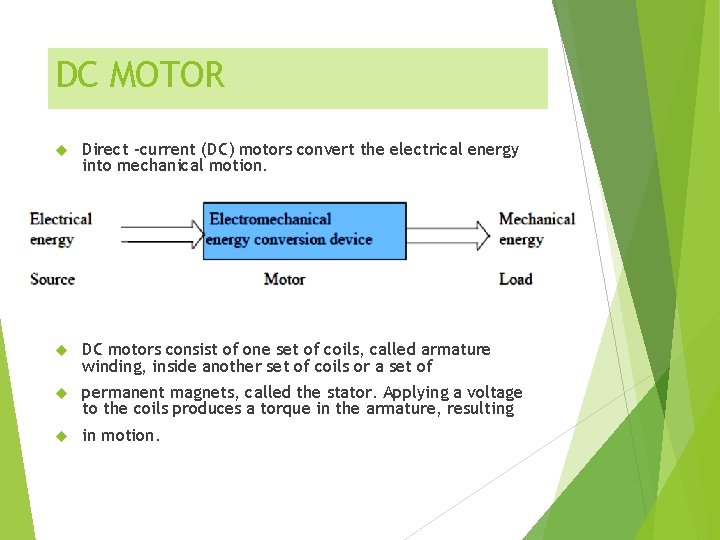
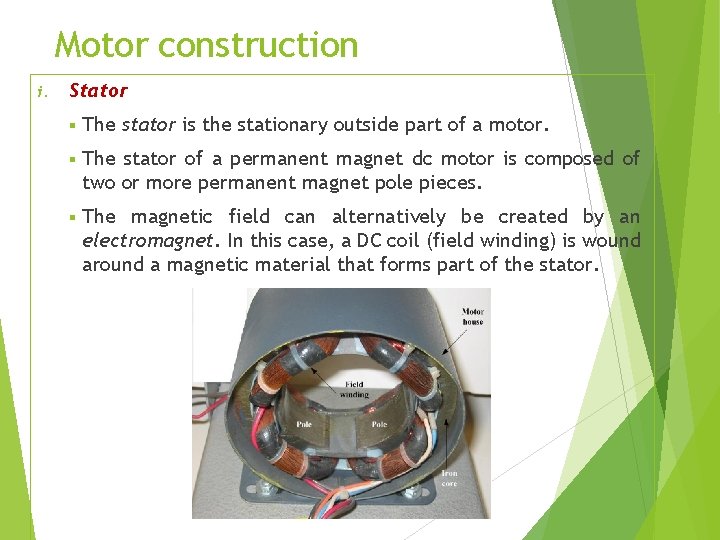
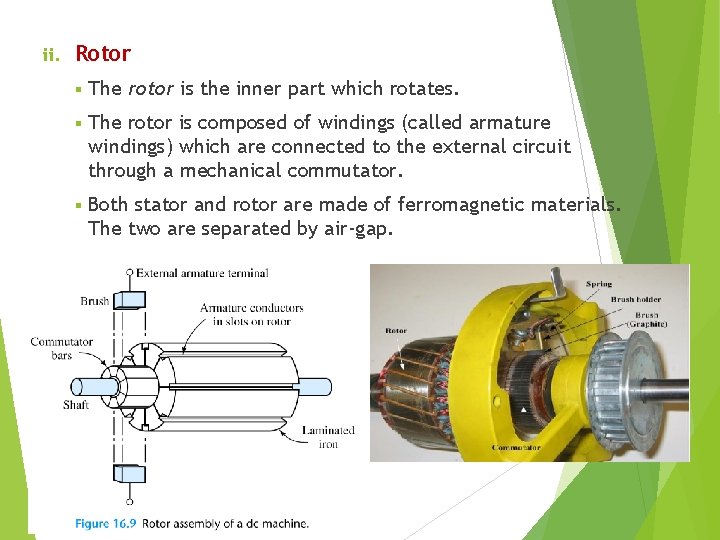
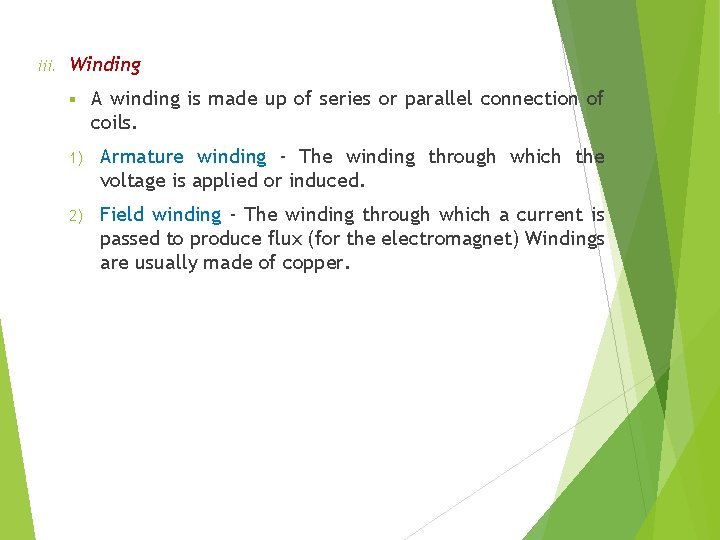
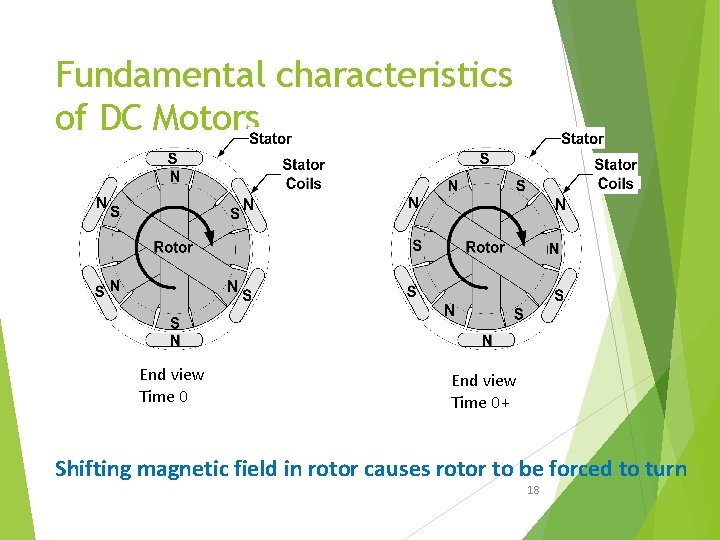
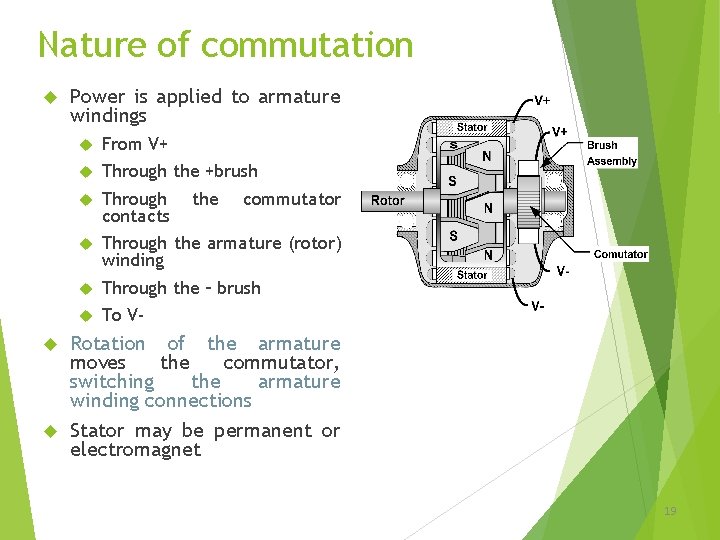
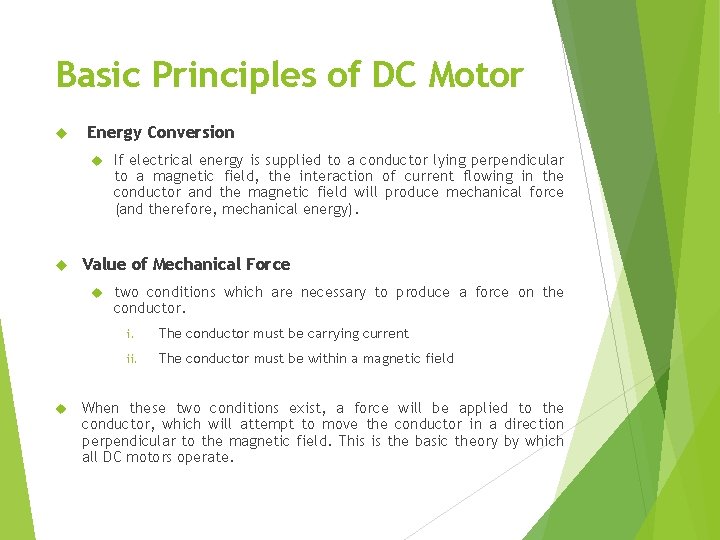
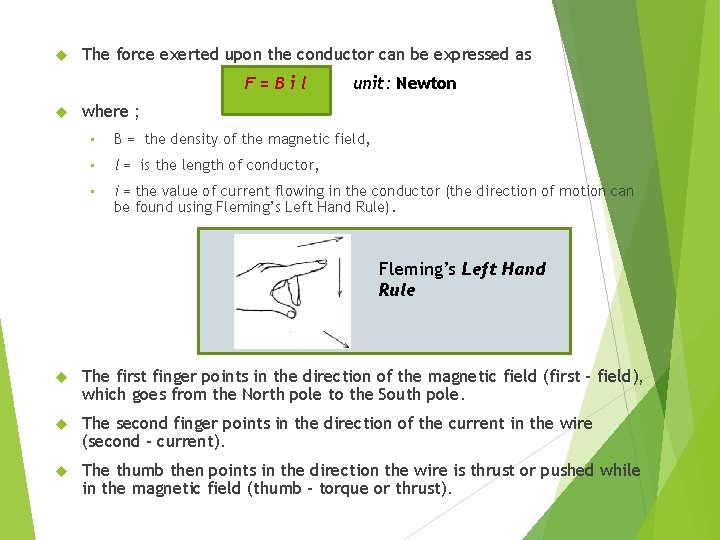
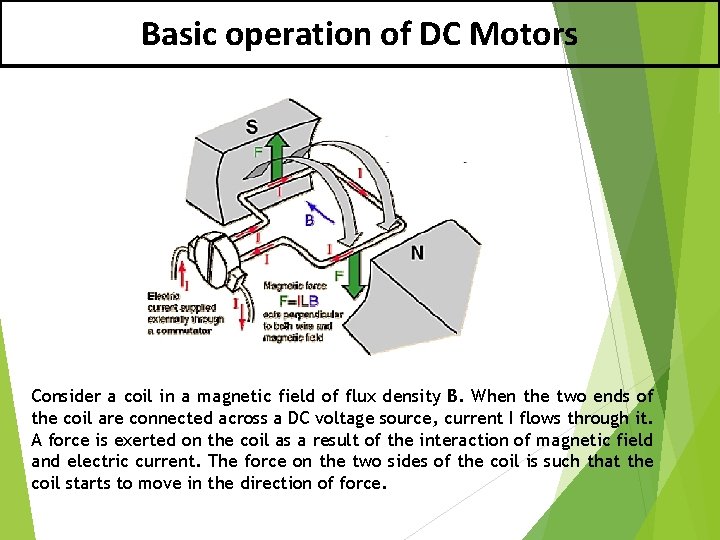
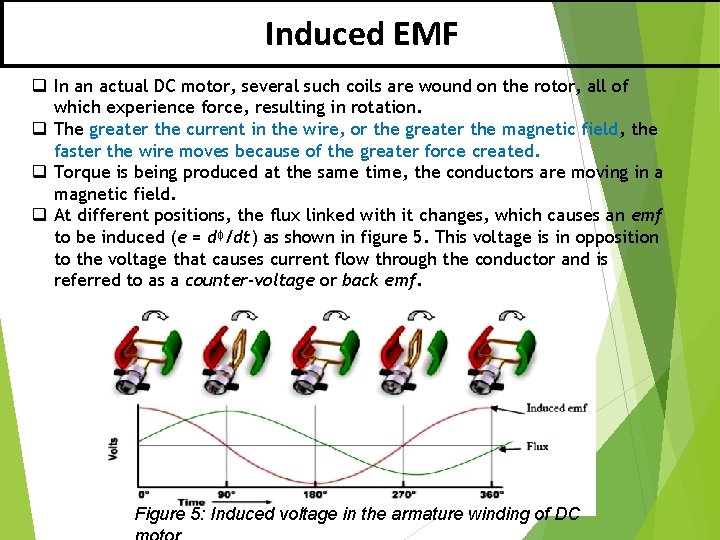
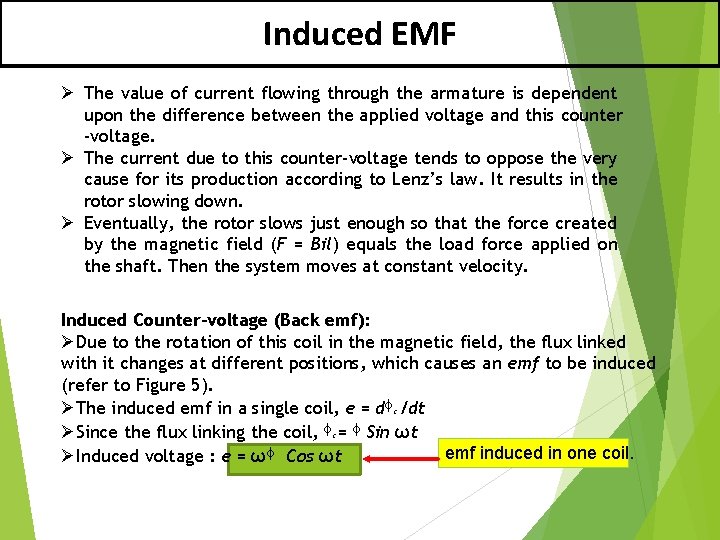
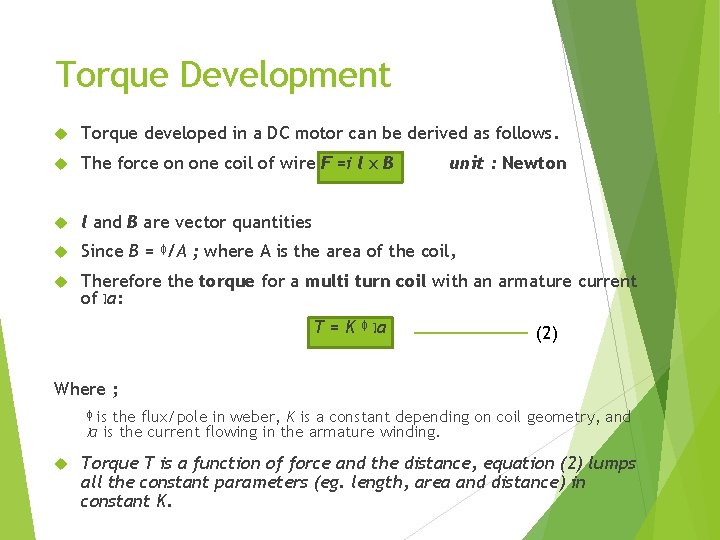
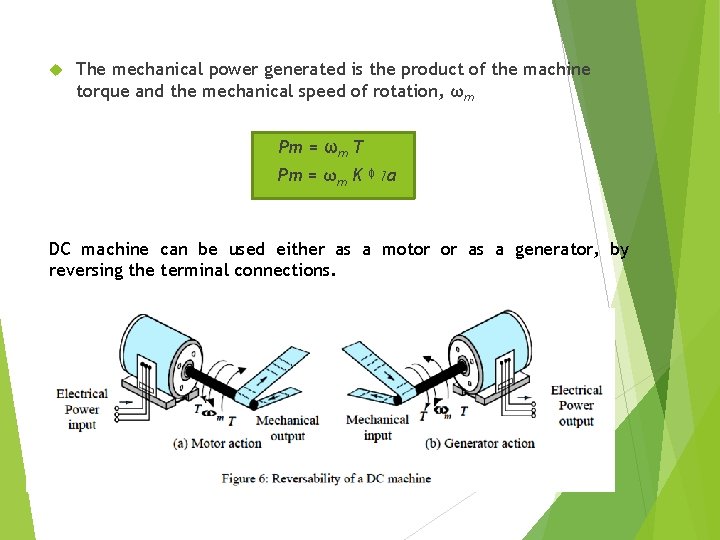
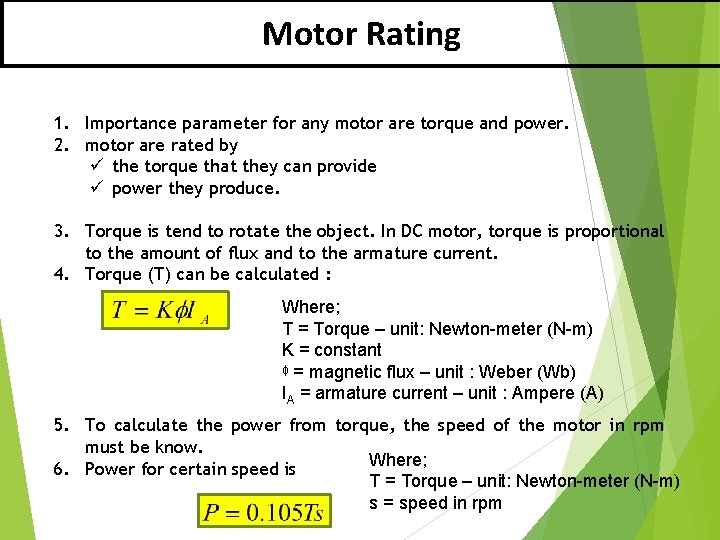
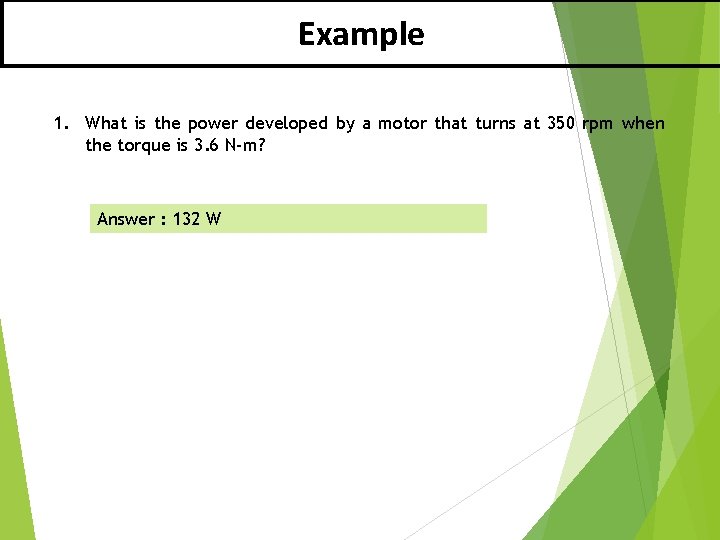
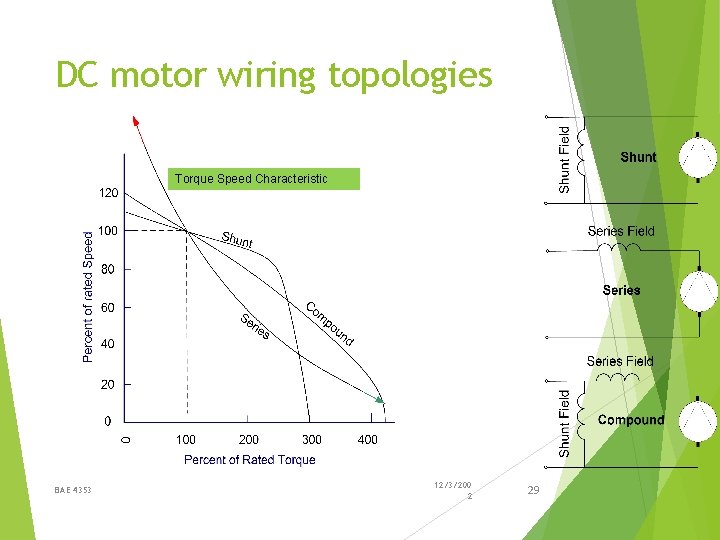
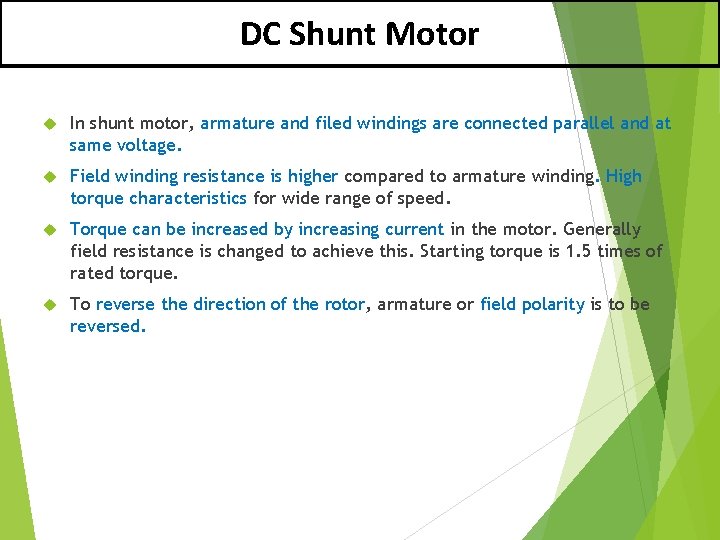
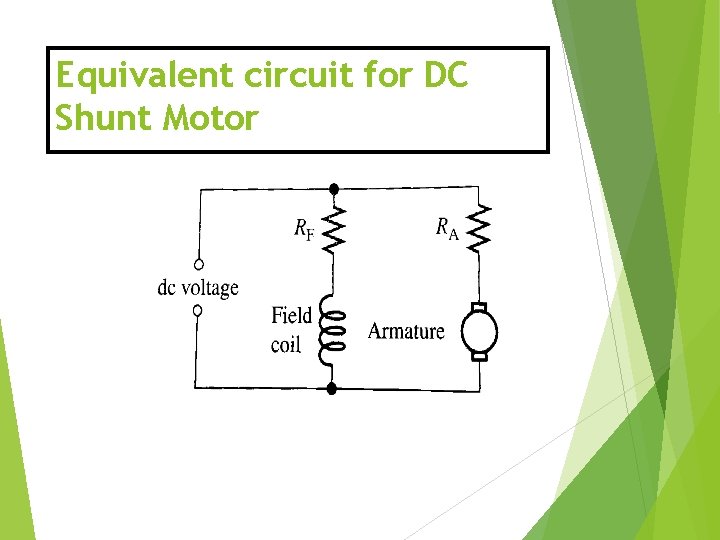
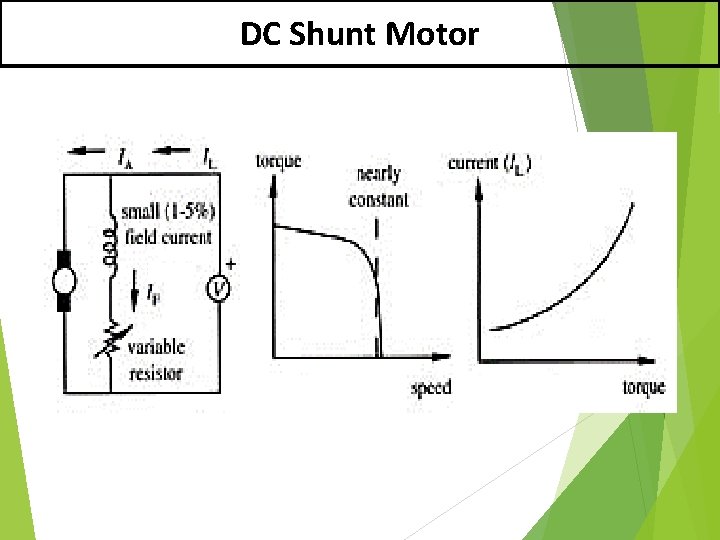
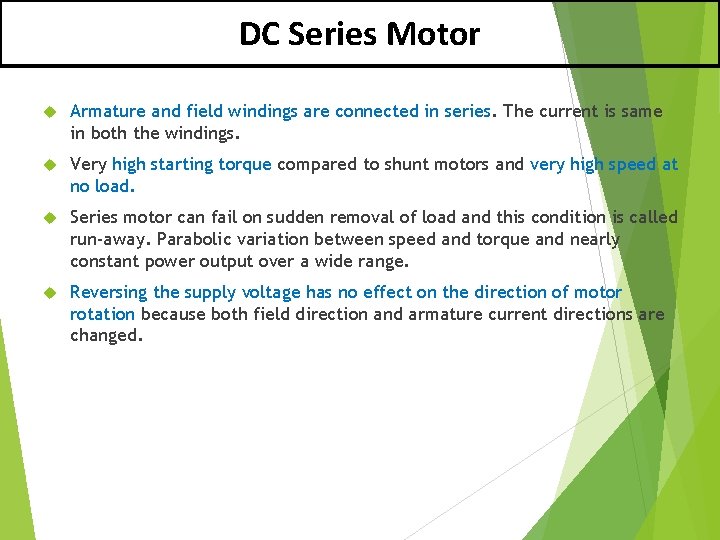
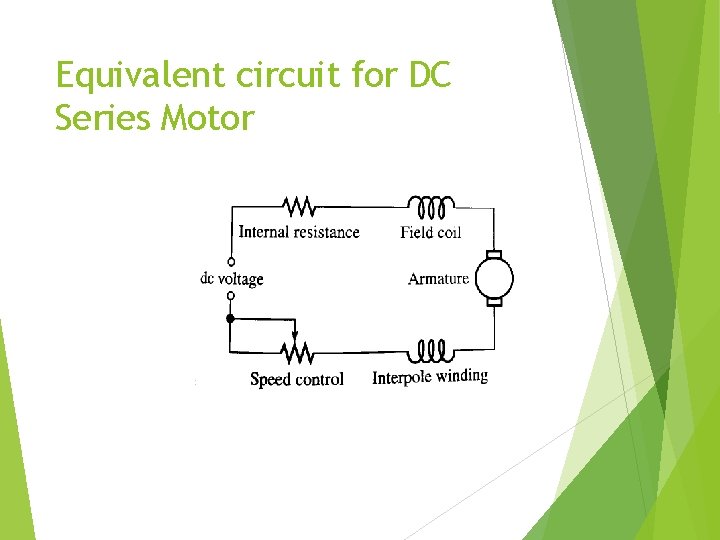
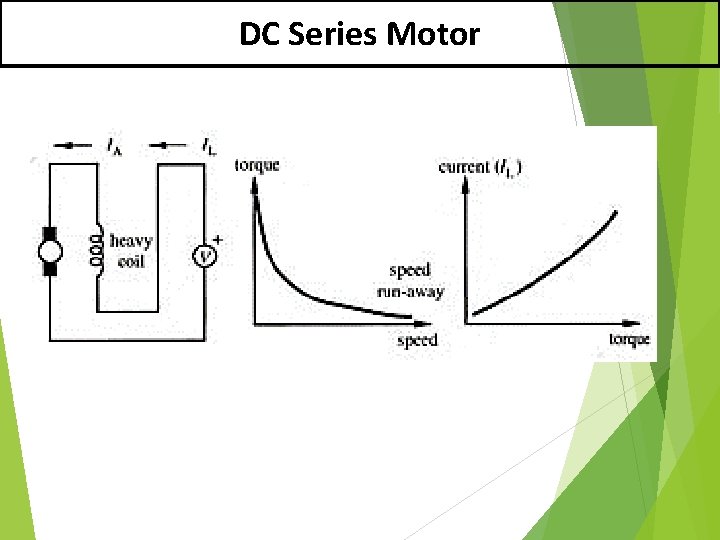
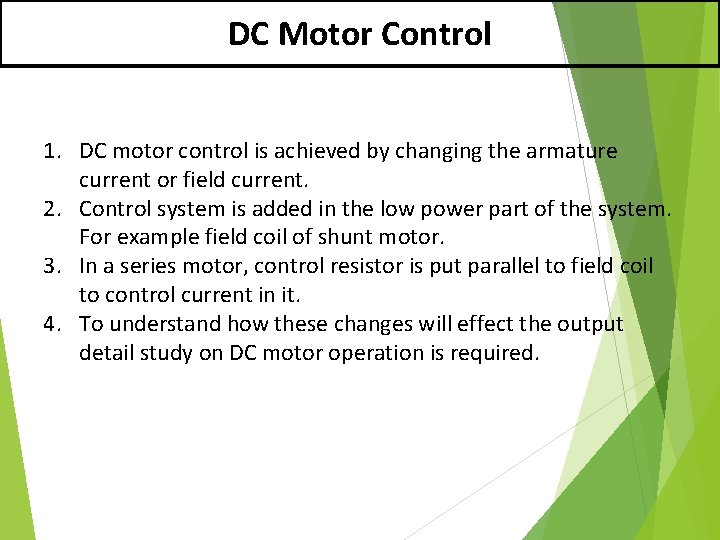
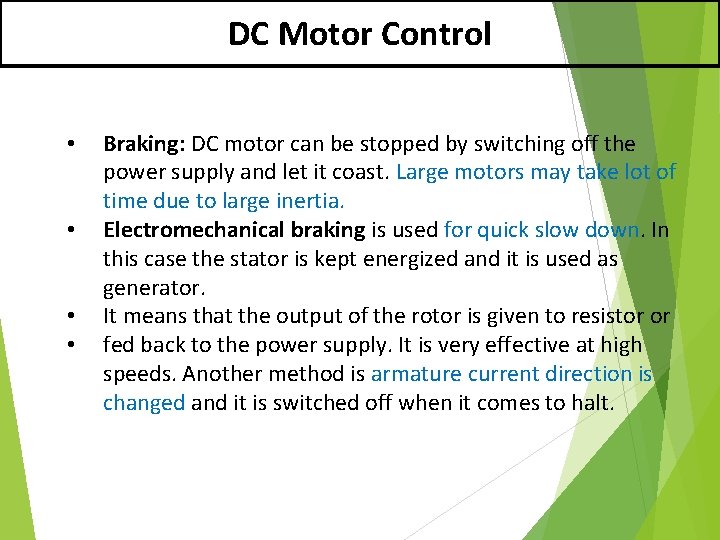
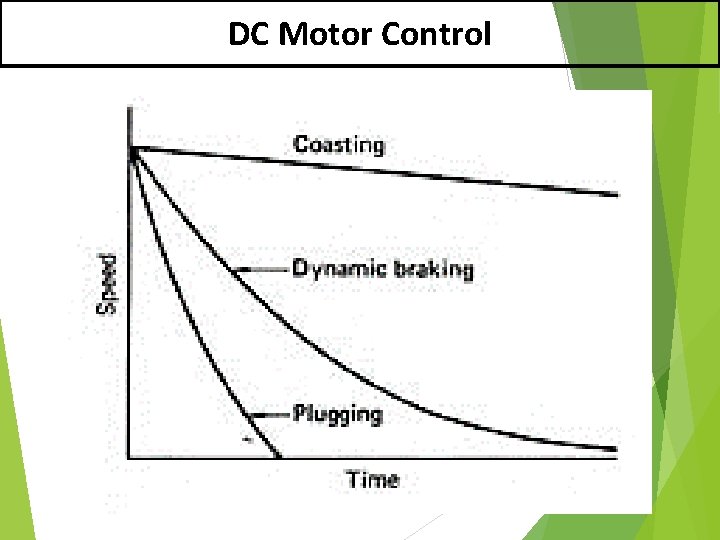
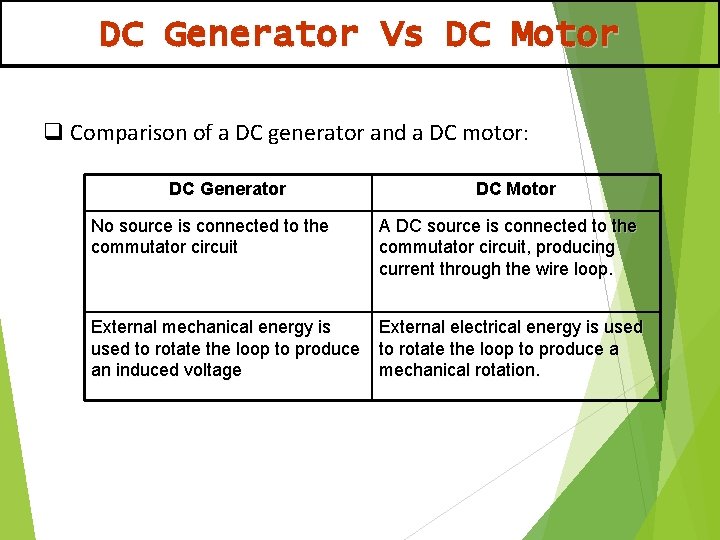
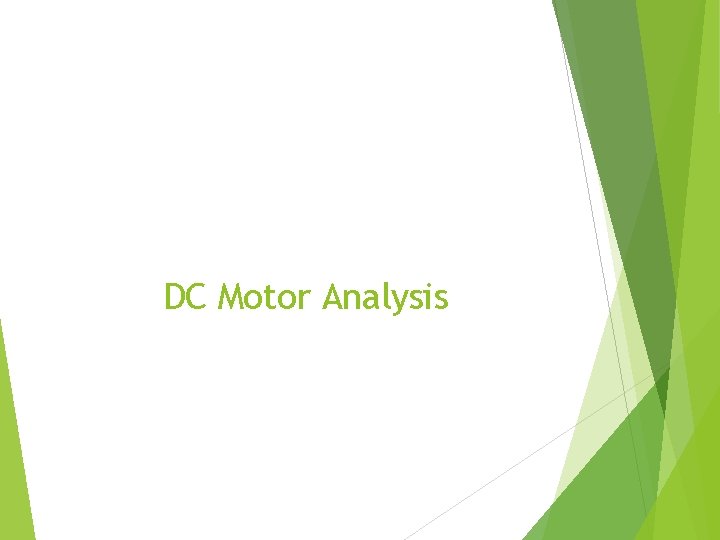
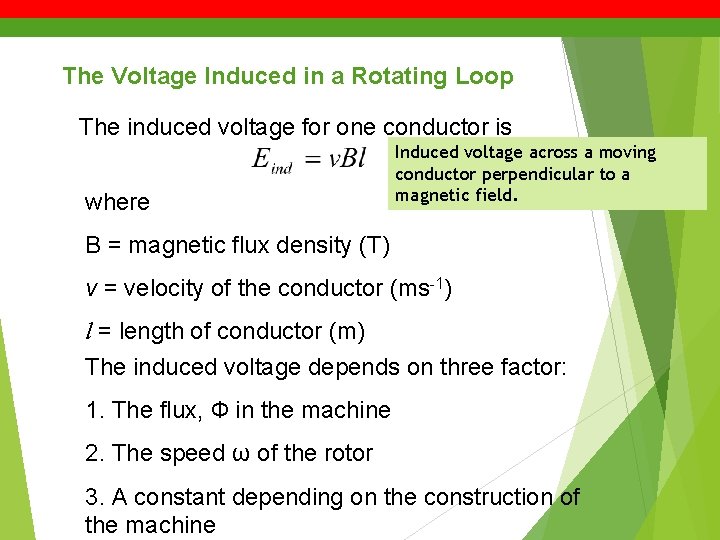
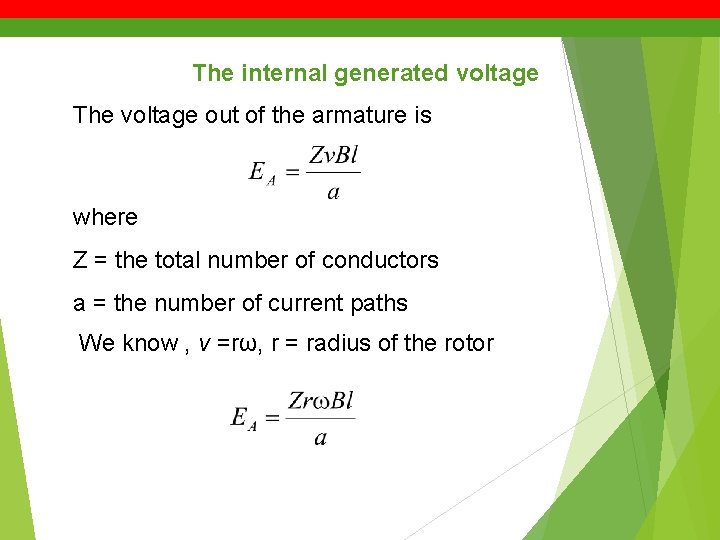
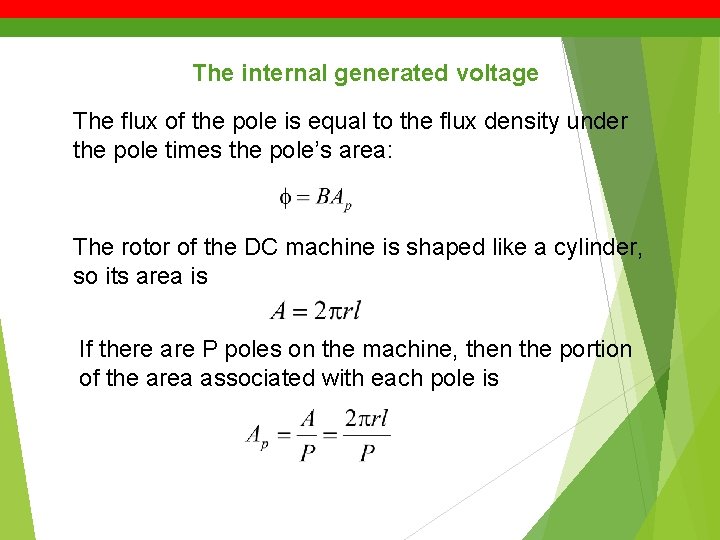
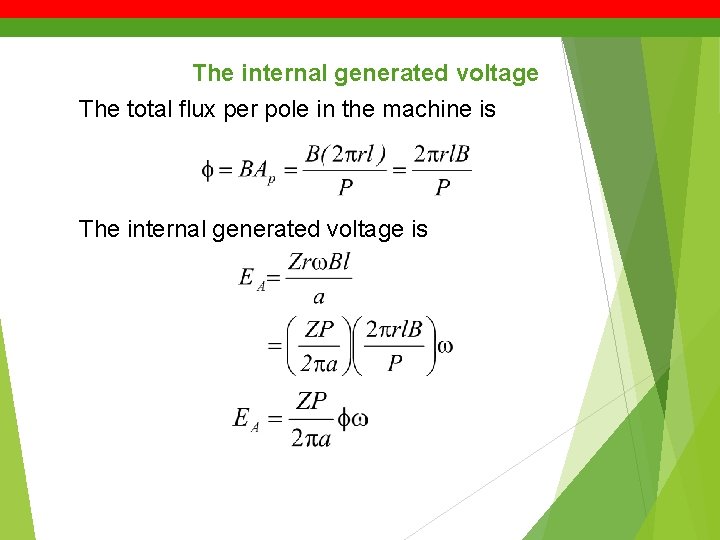
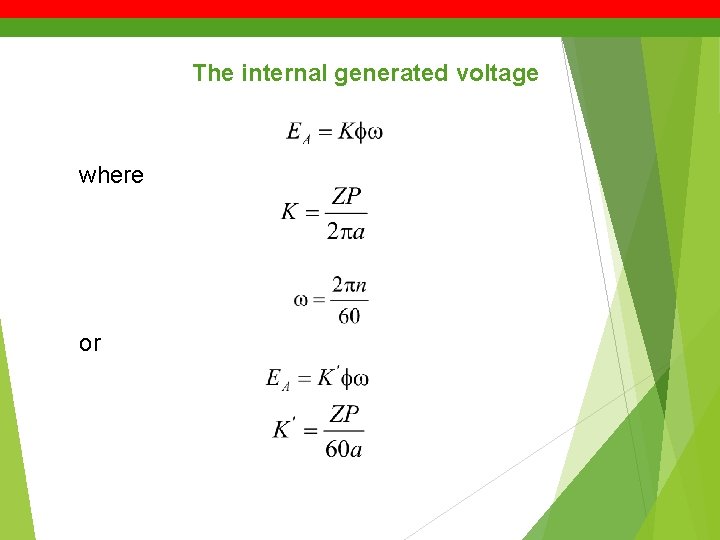
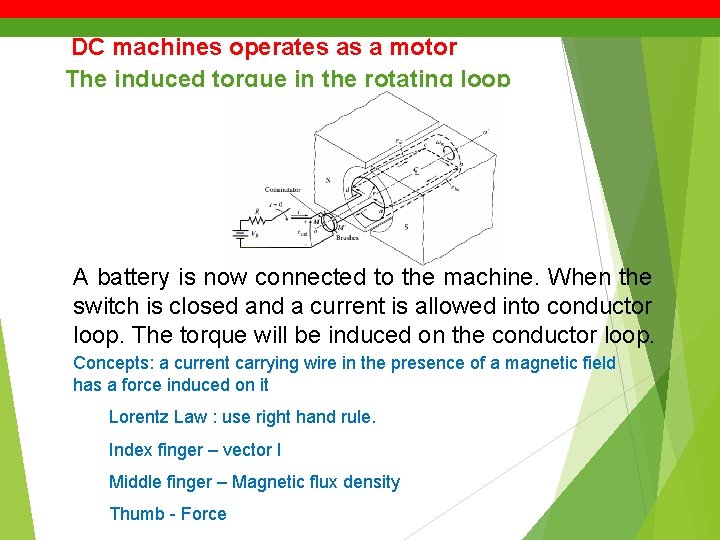
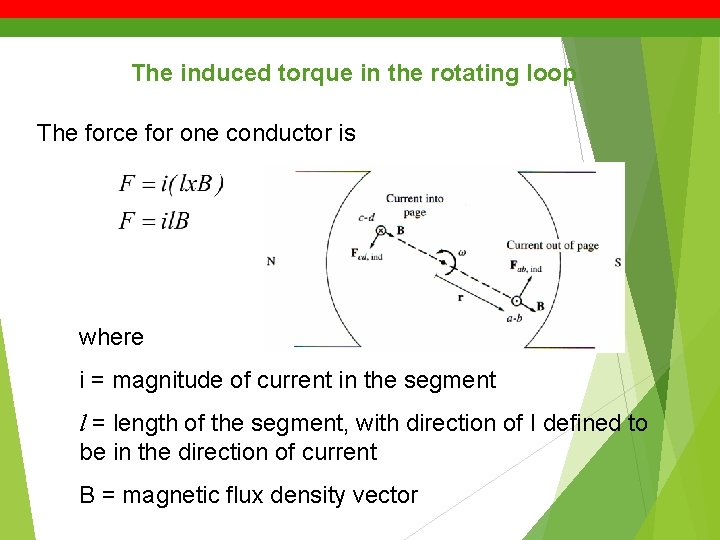
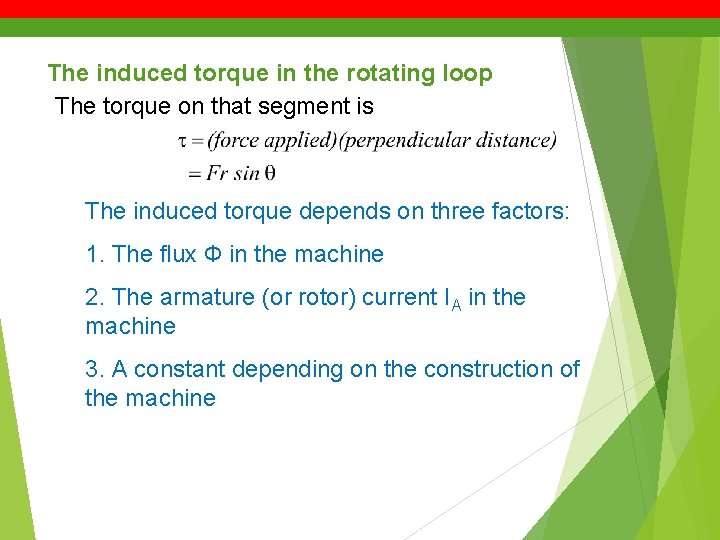
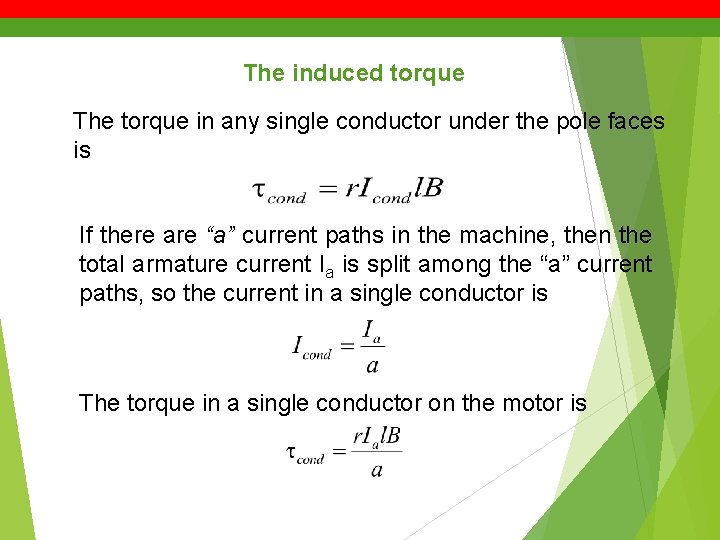
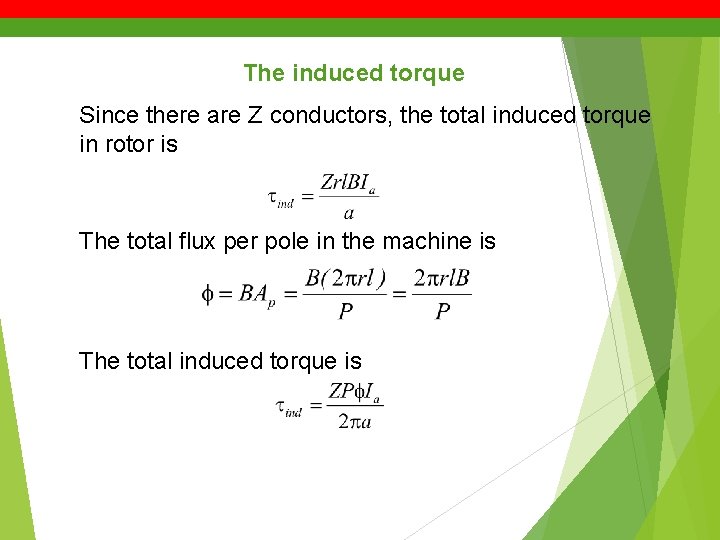
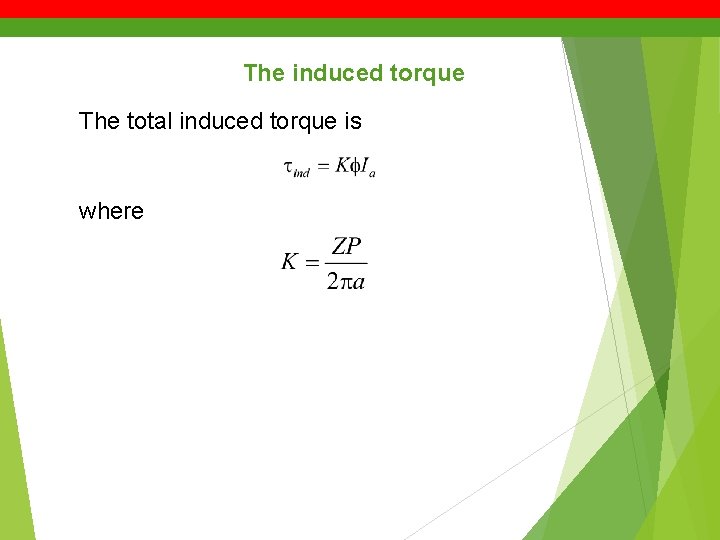
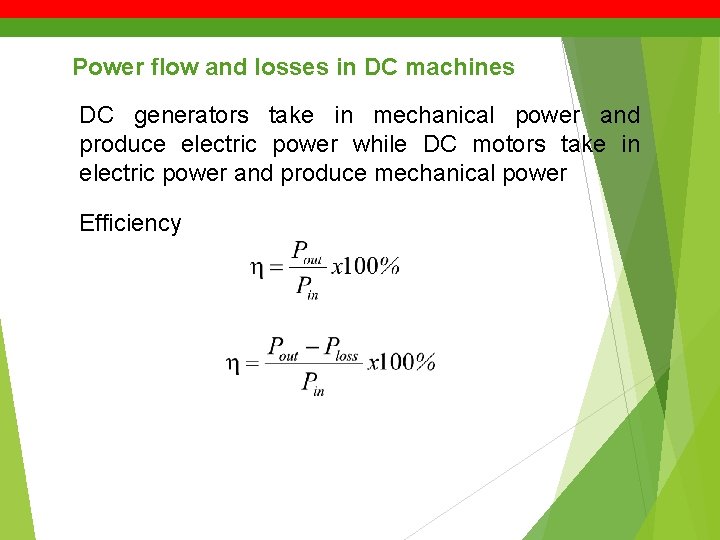
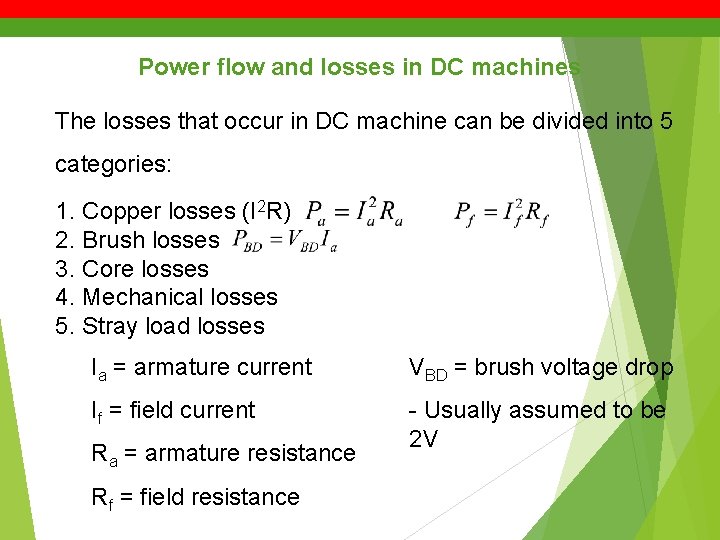
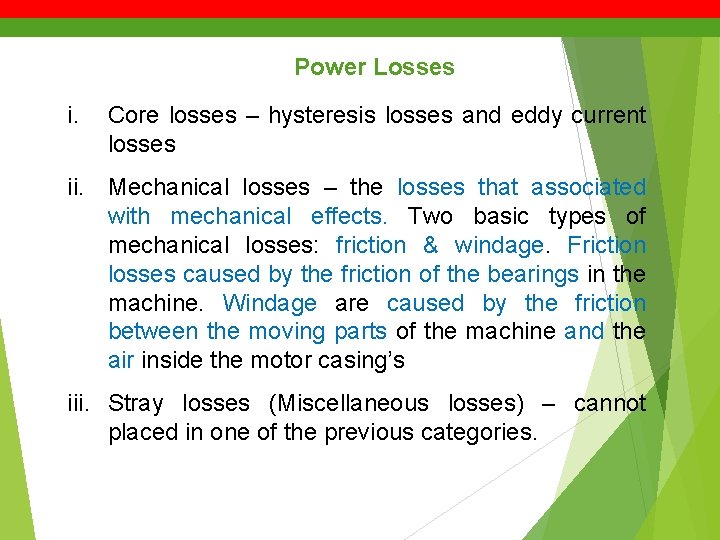
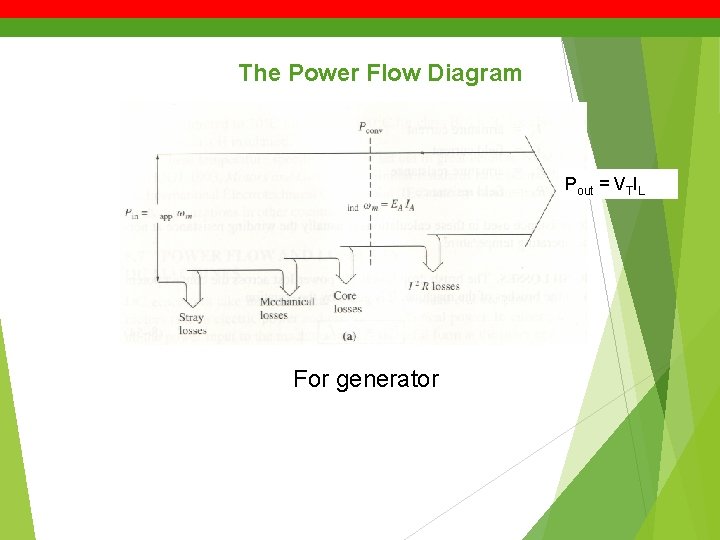
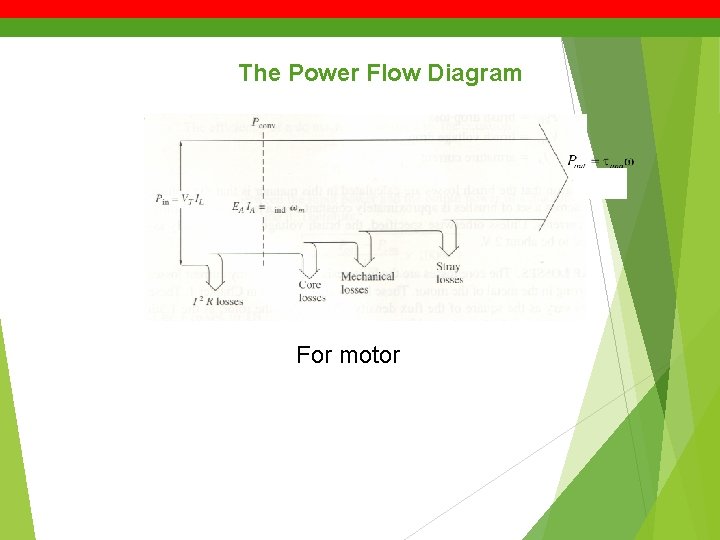
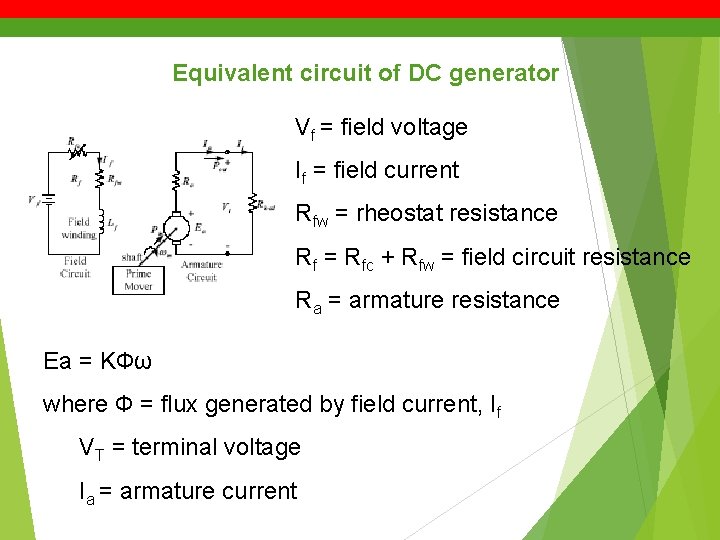
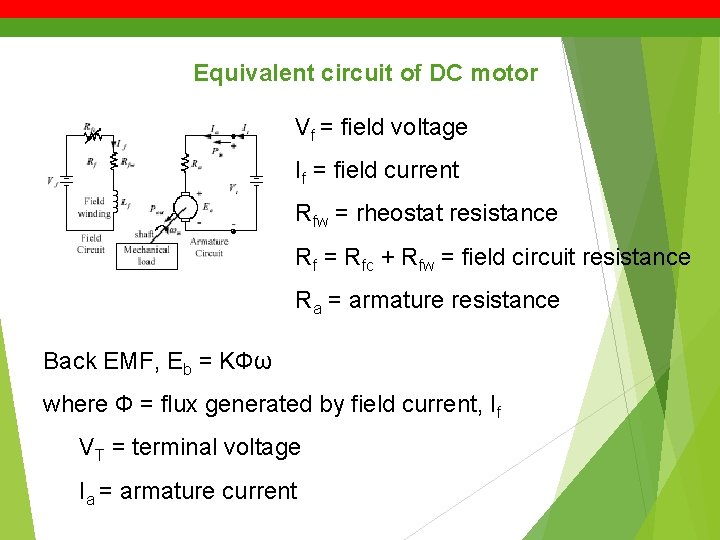
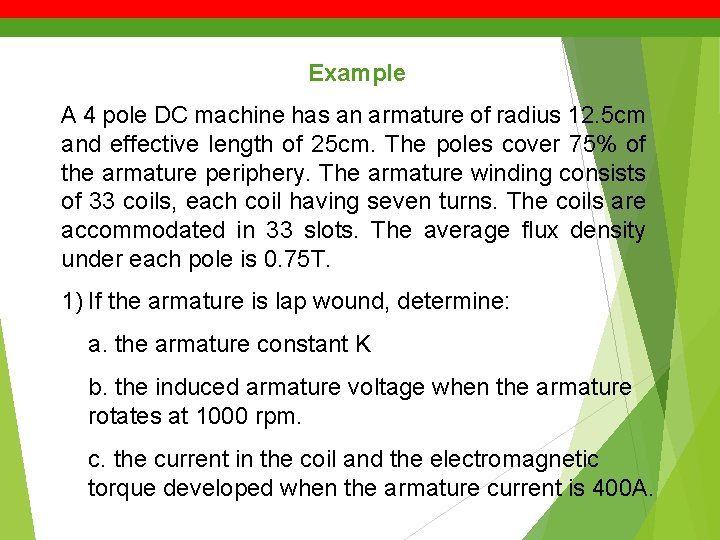
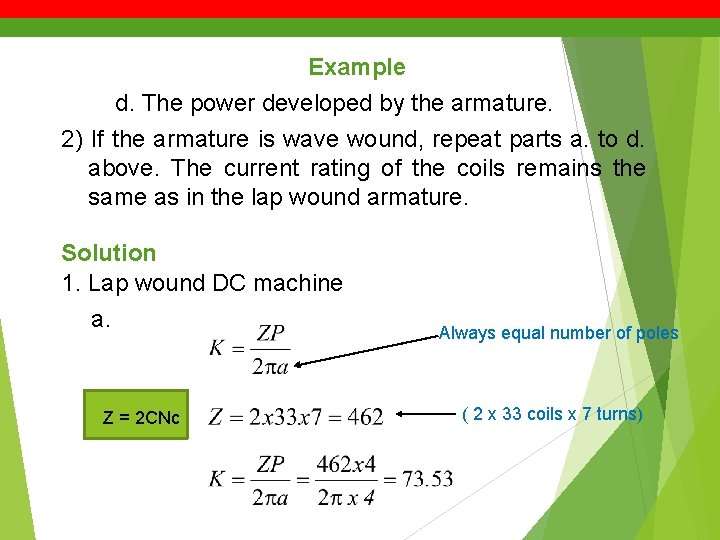
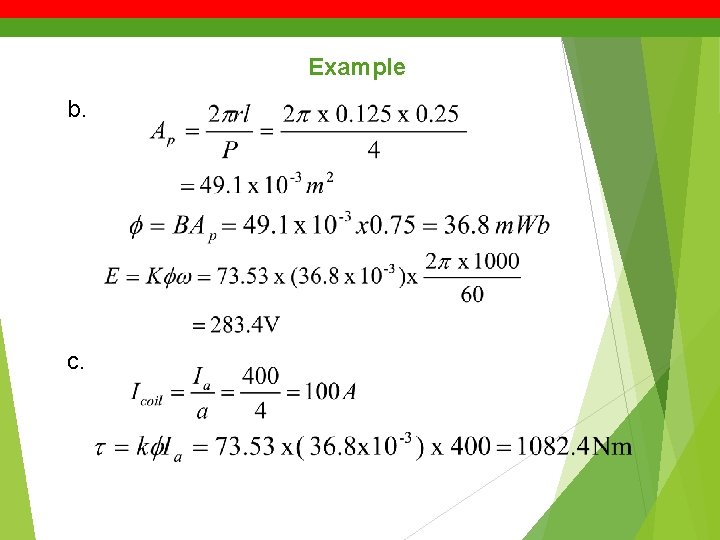
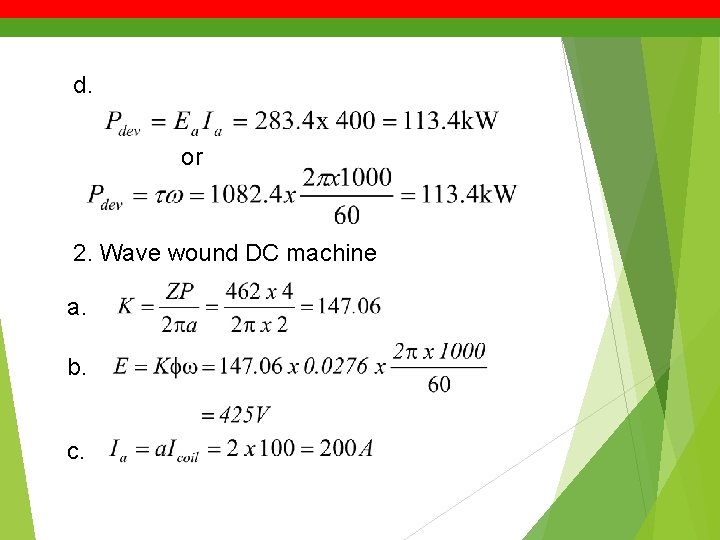
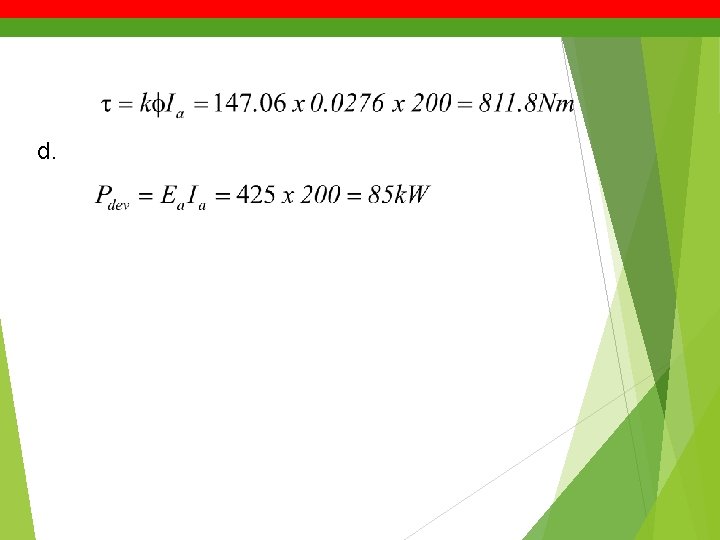
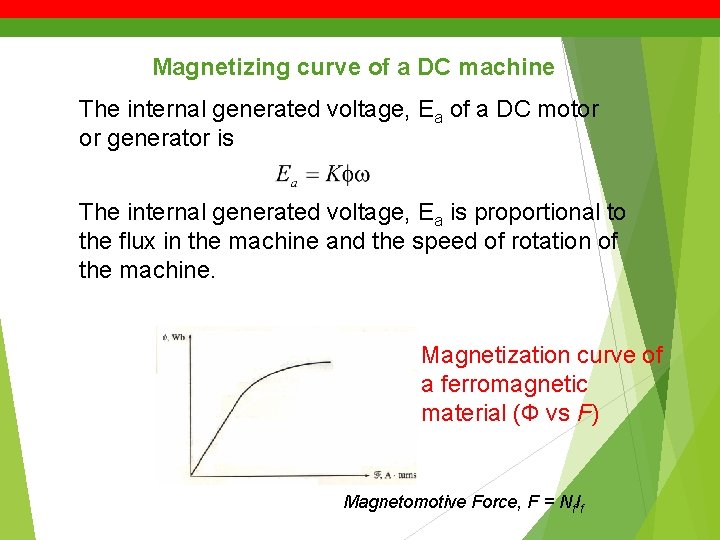
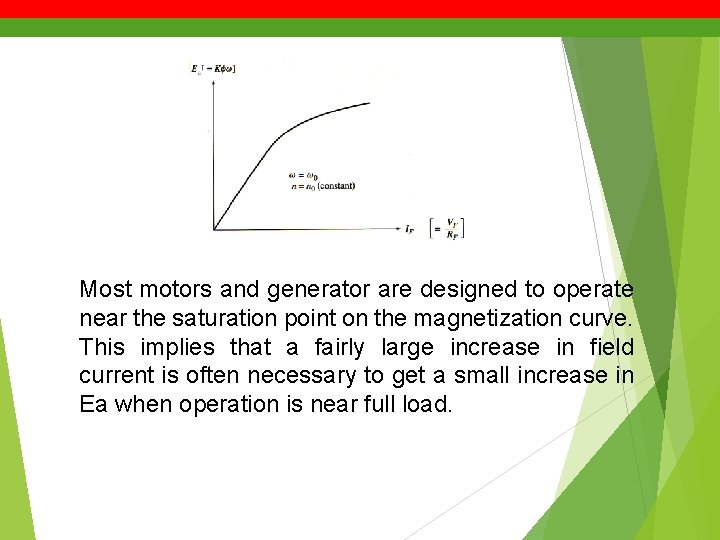
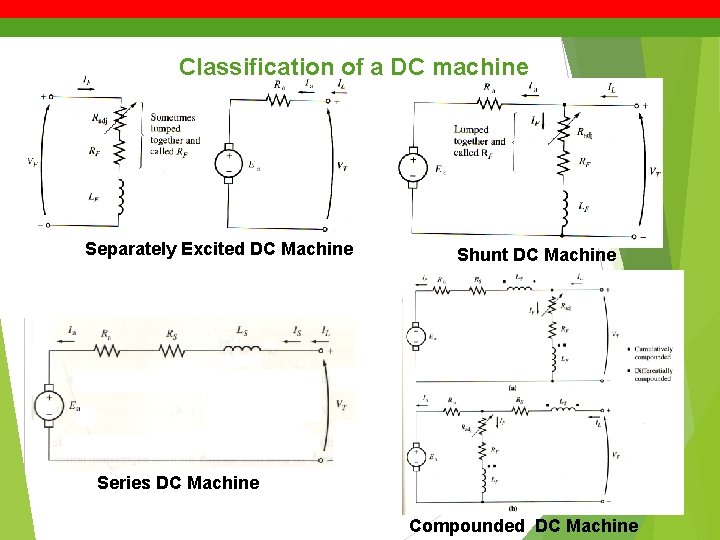
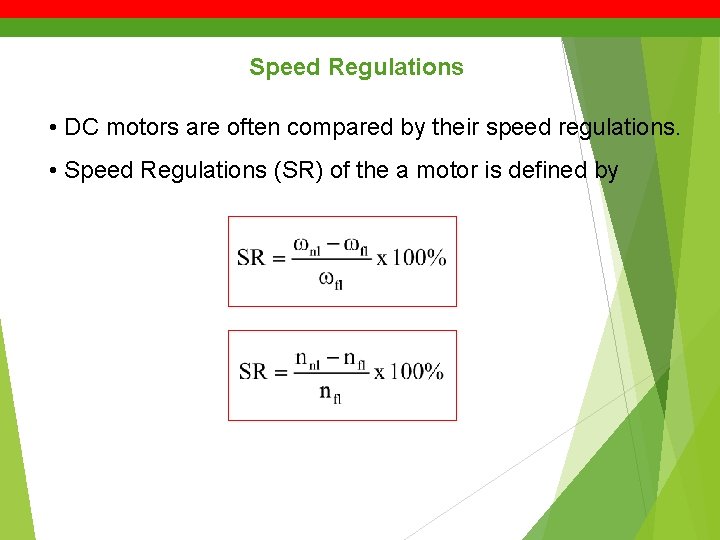
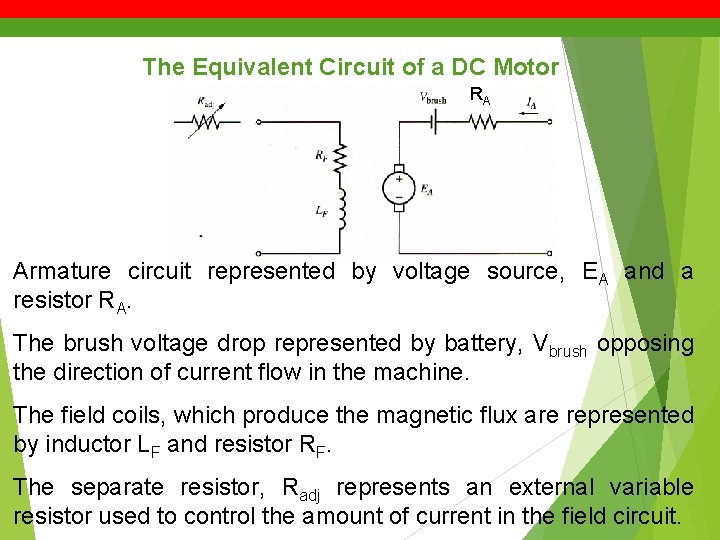
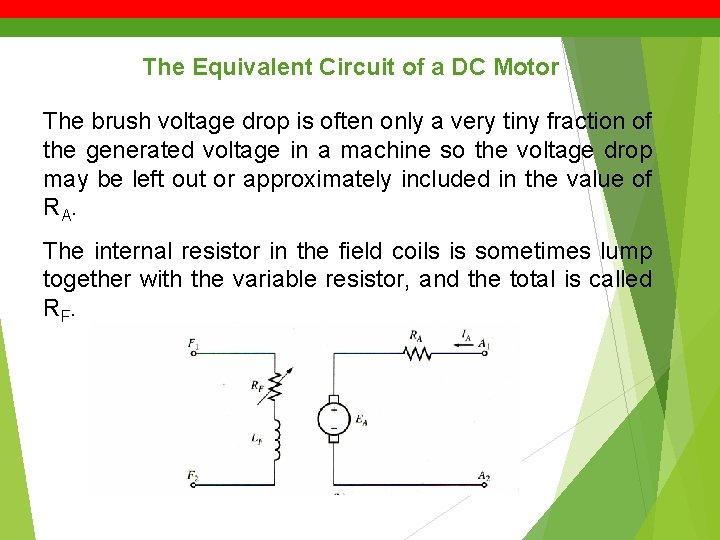
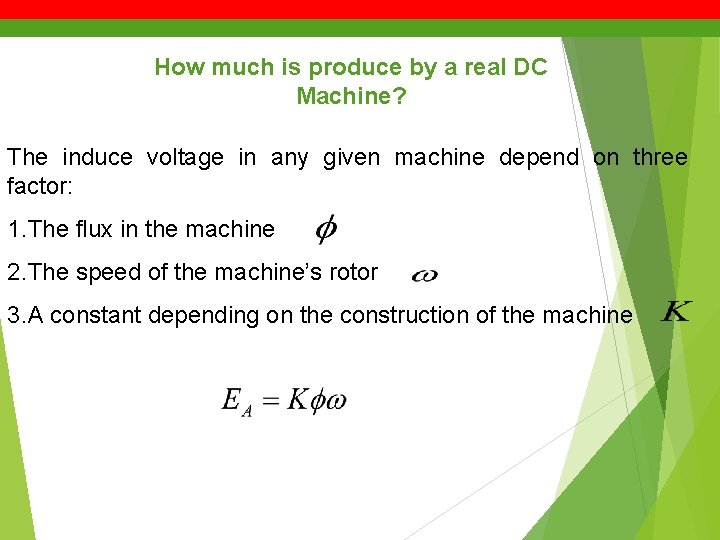
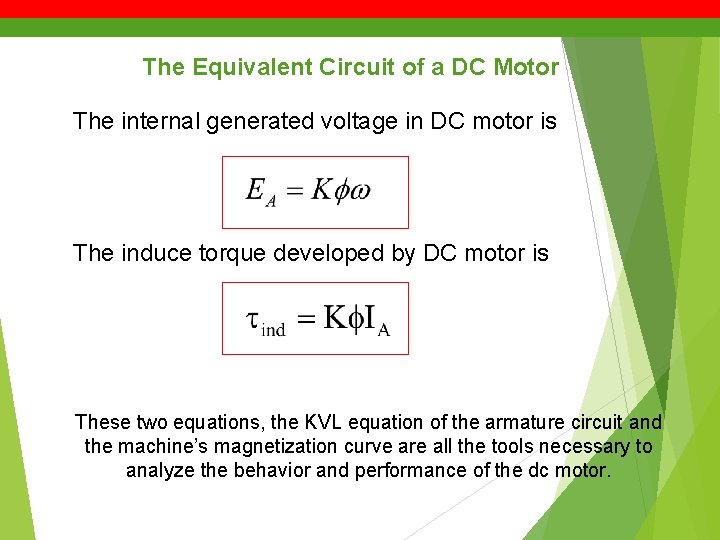
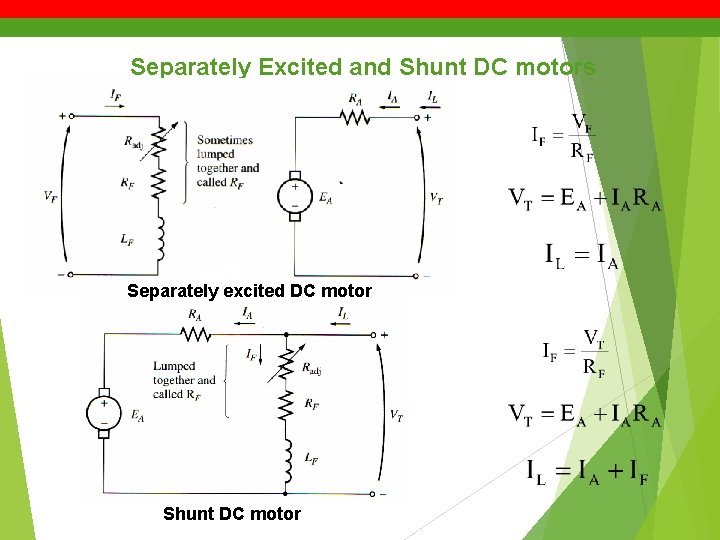
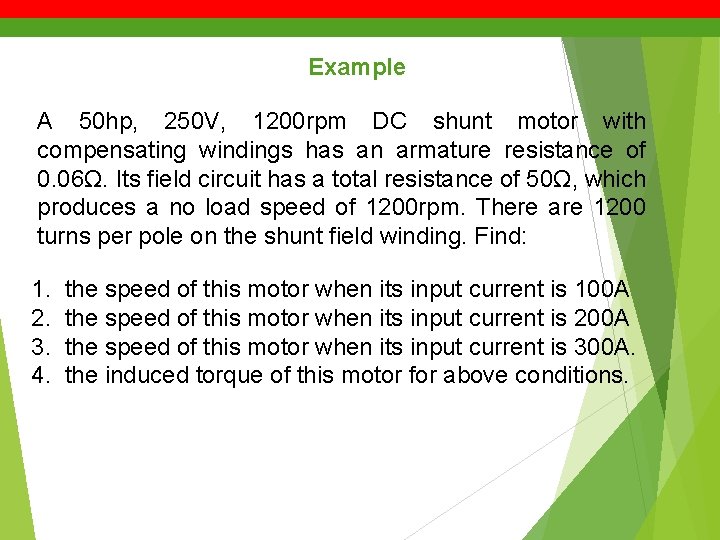
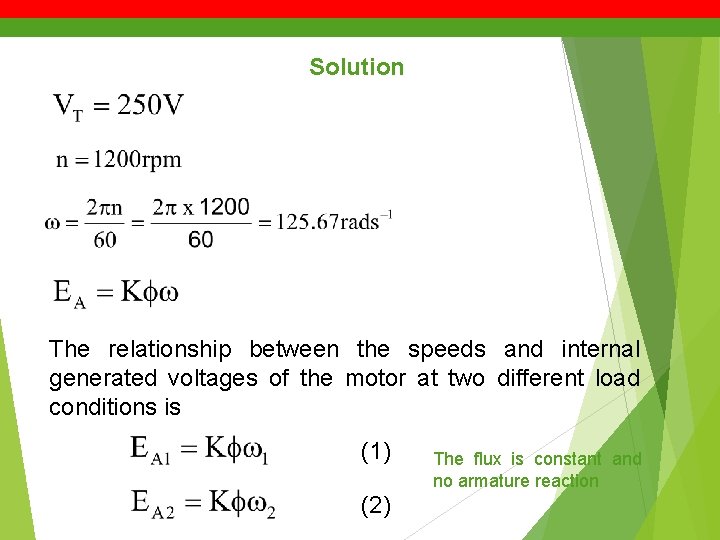
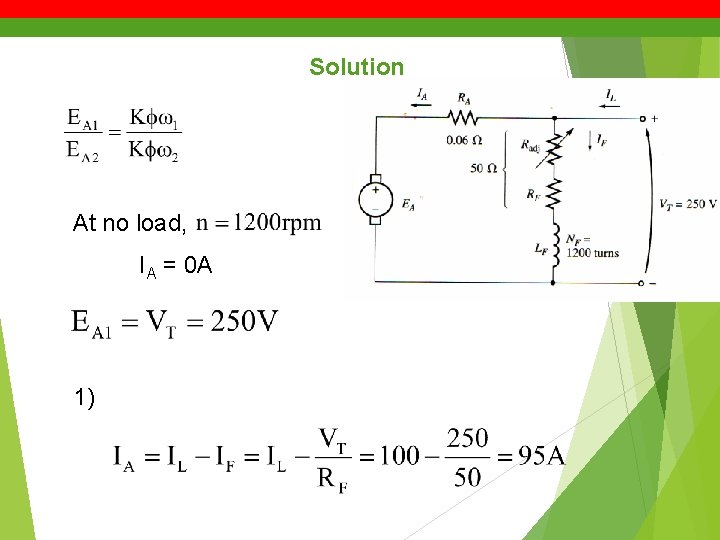
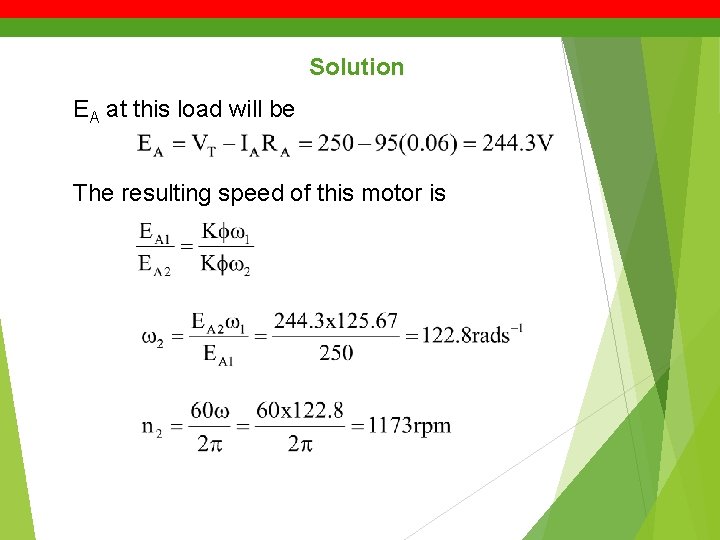
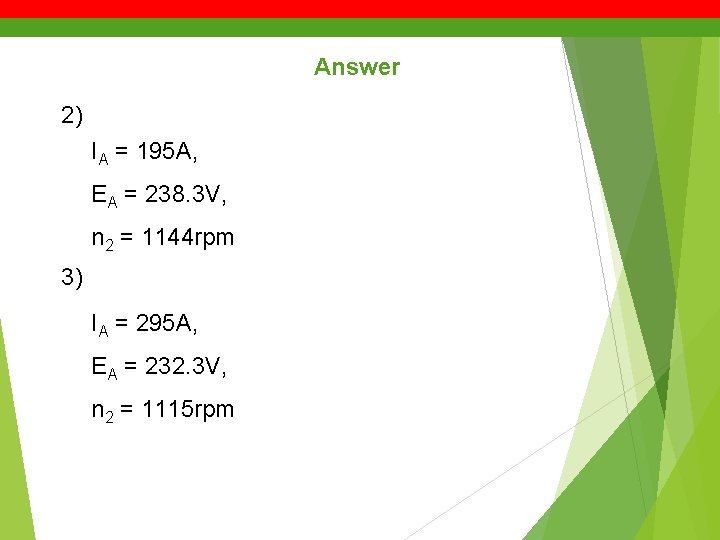
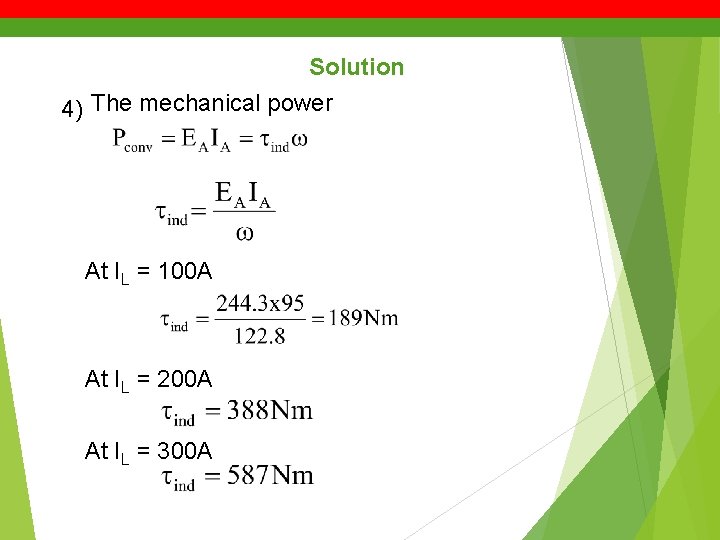
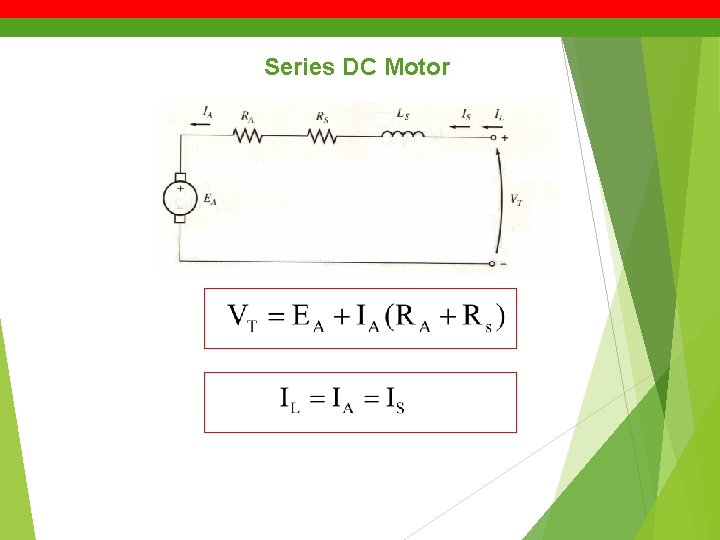
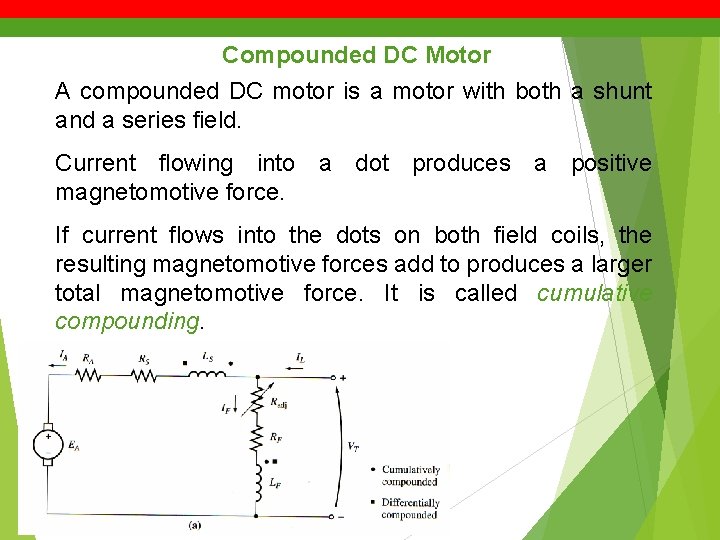
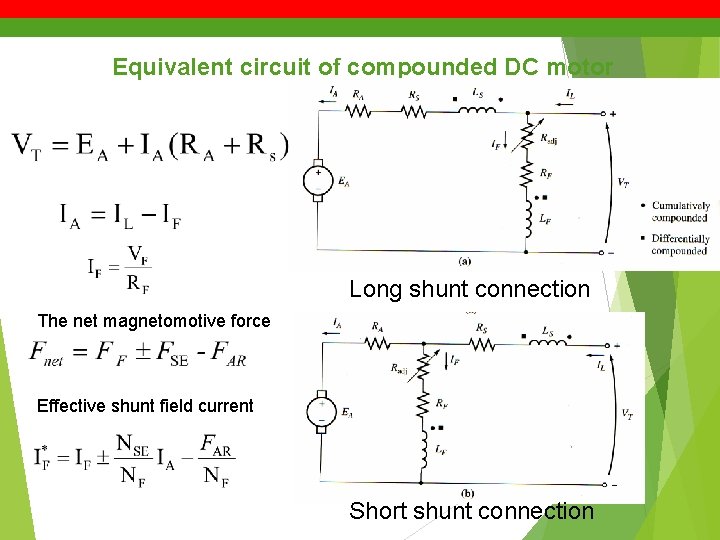
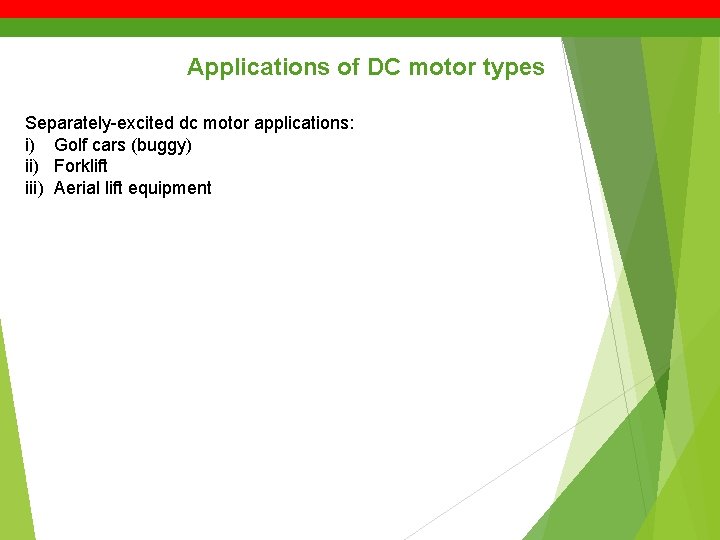
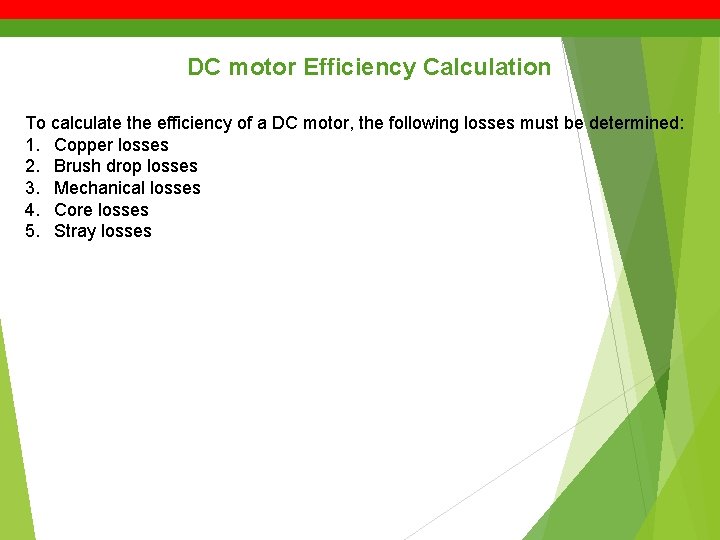
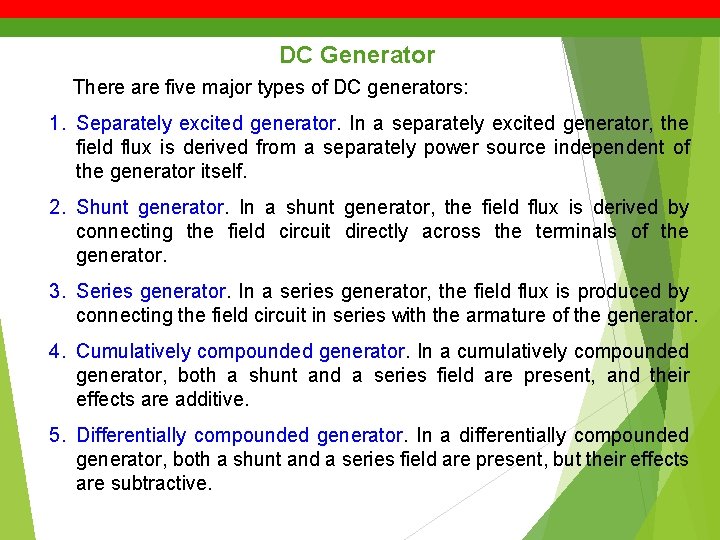
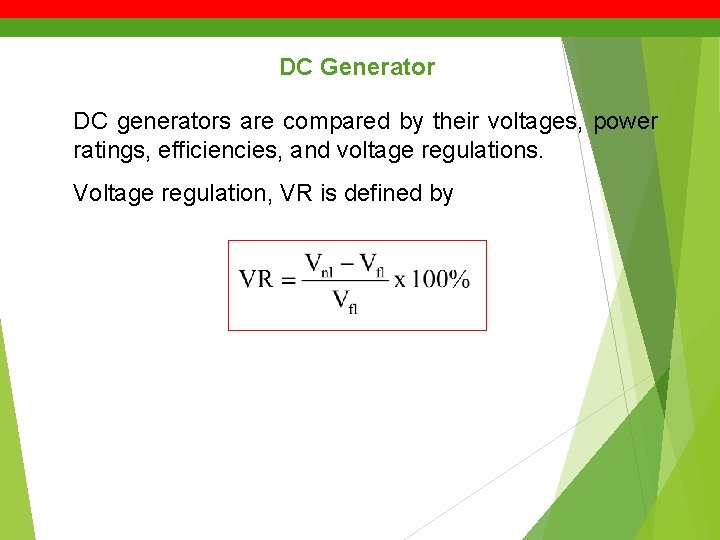
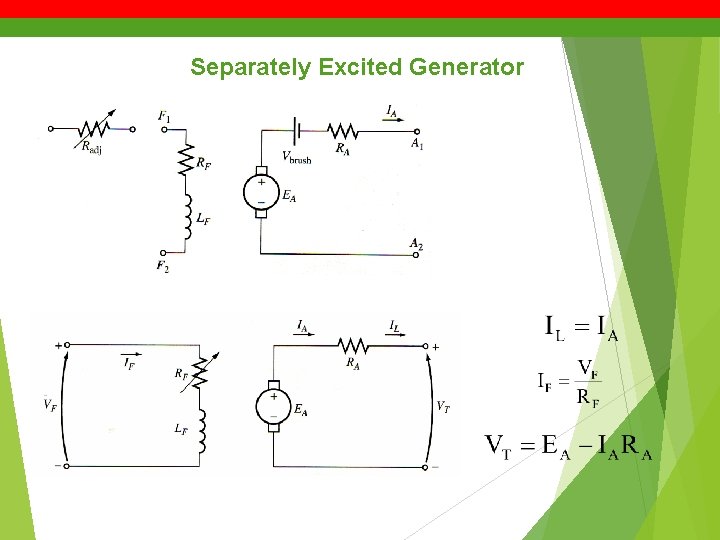
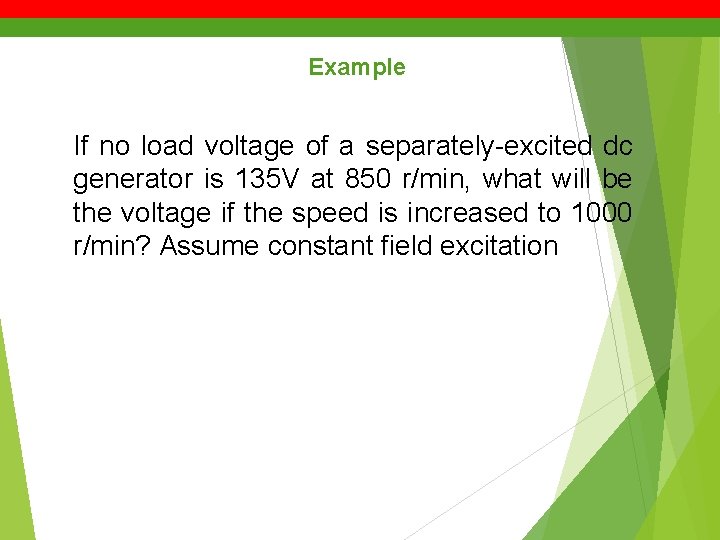
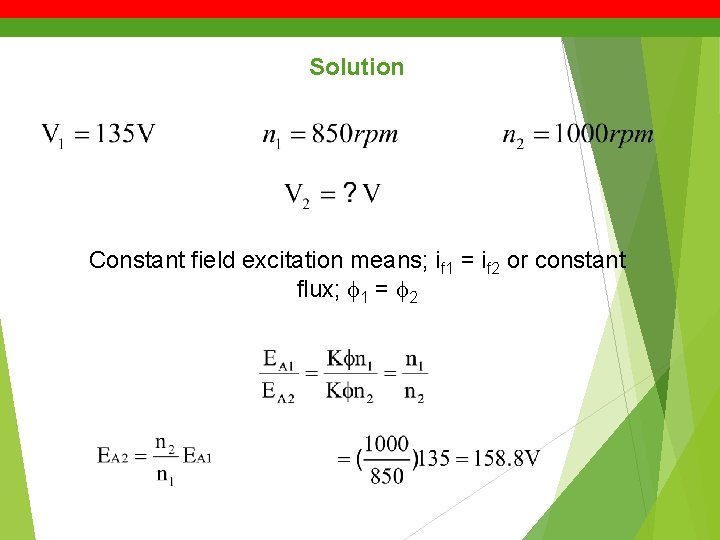
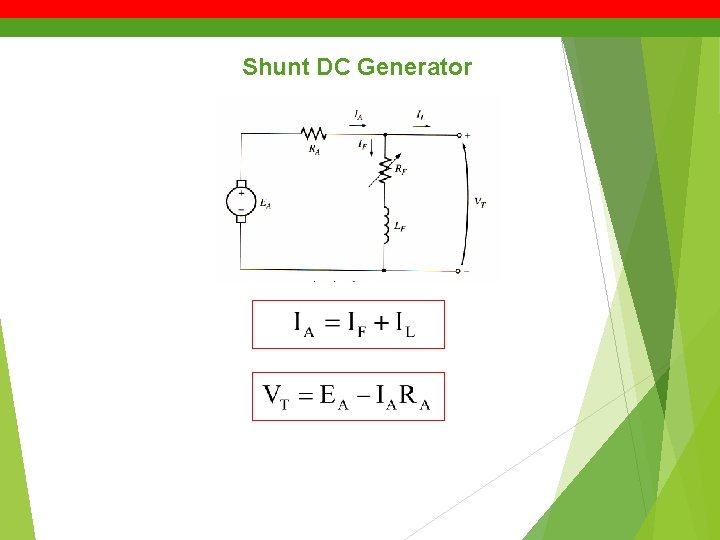
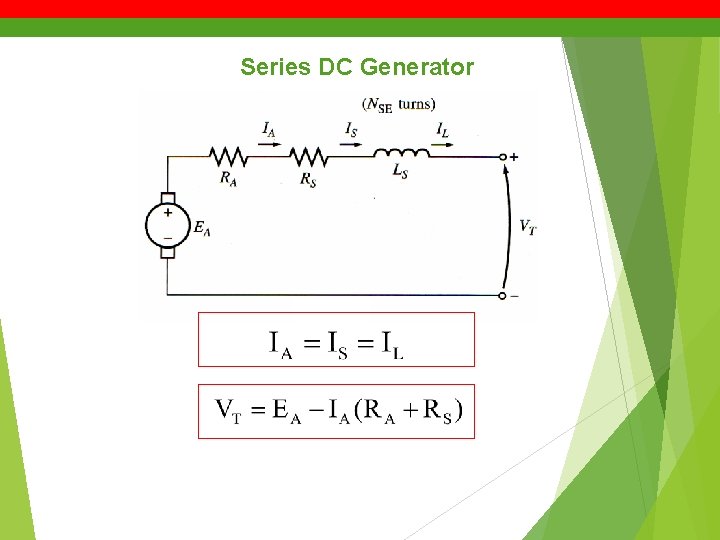
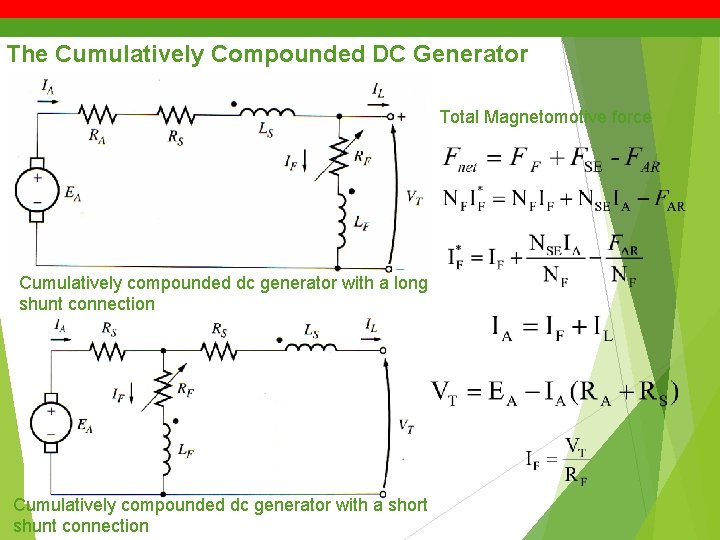
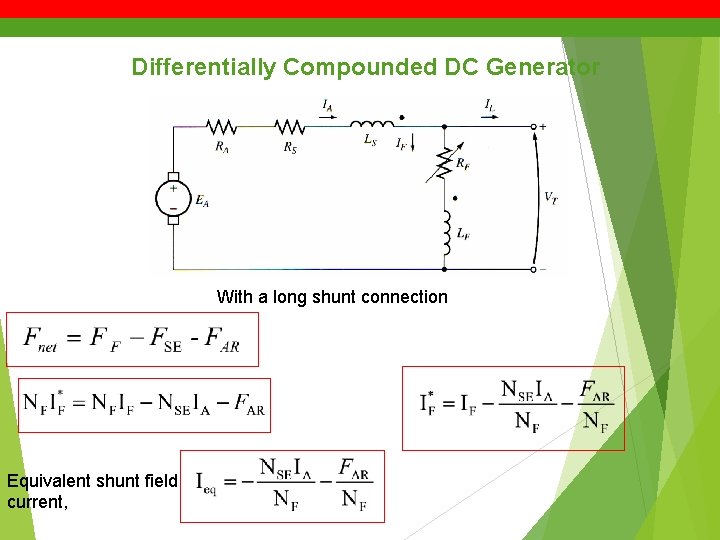
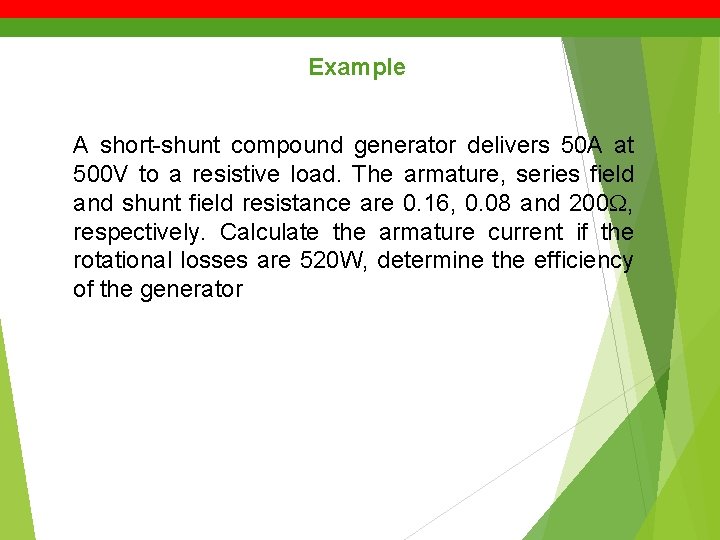
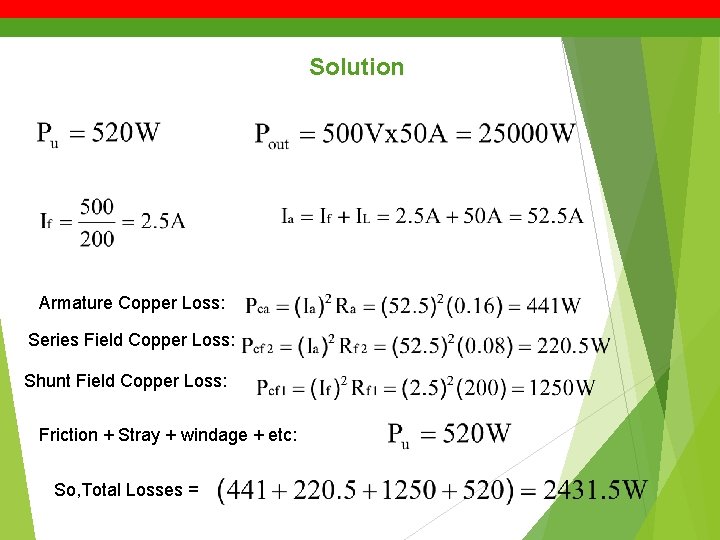
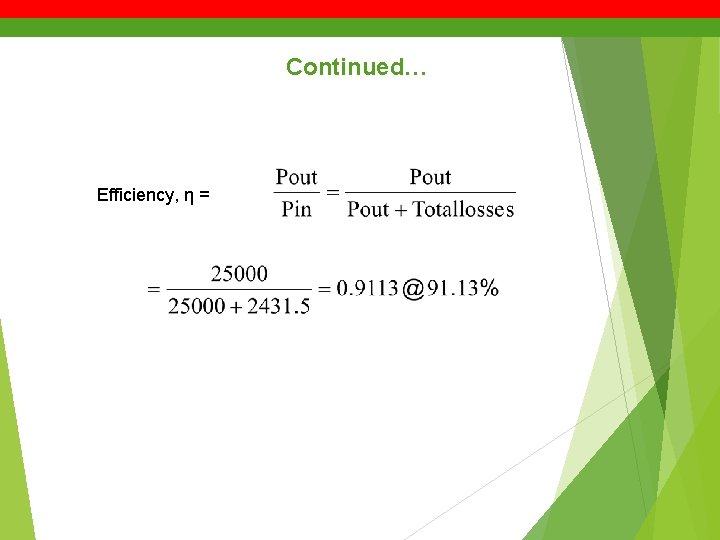
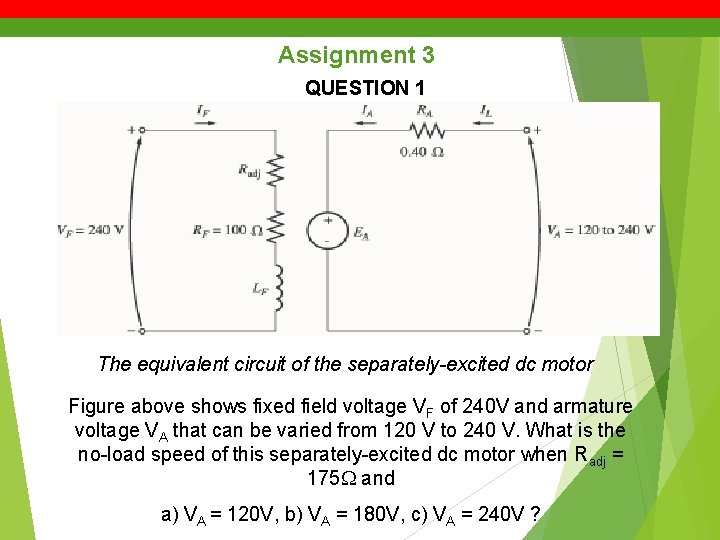
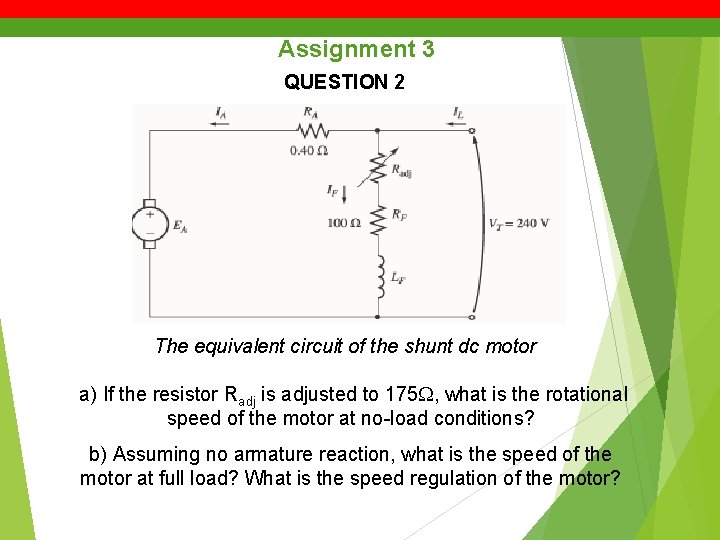
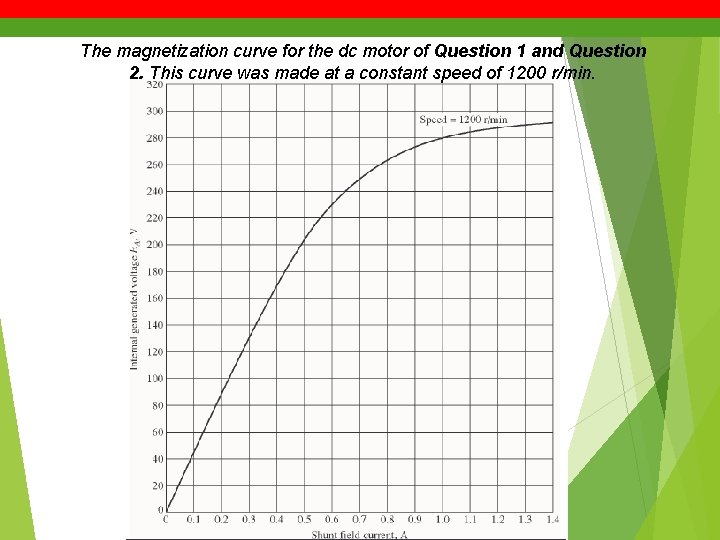
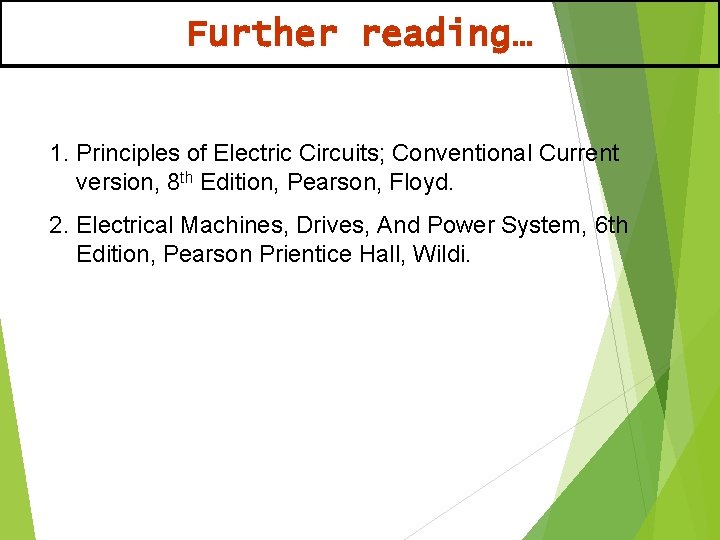
- Slides: 99
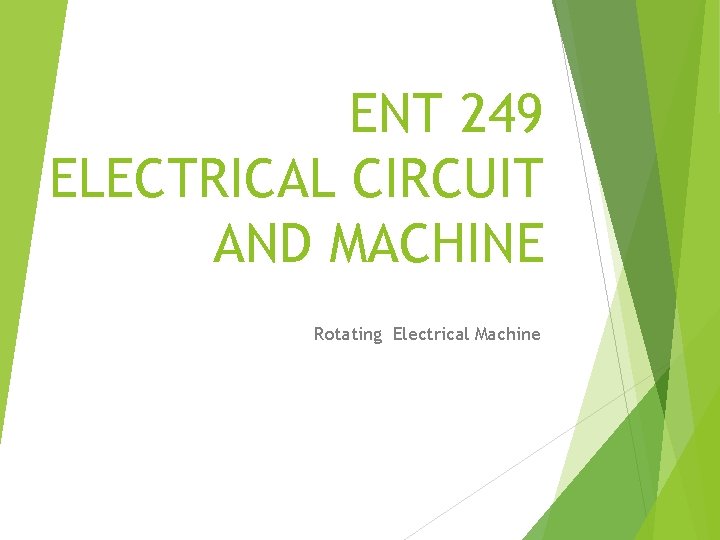
ENT 249 ELECTRICAL CIRCUIT AND MACHINE Rotating Electrical Machine
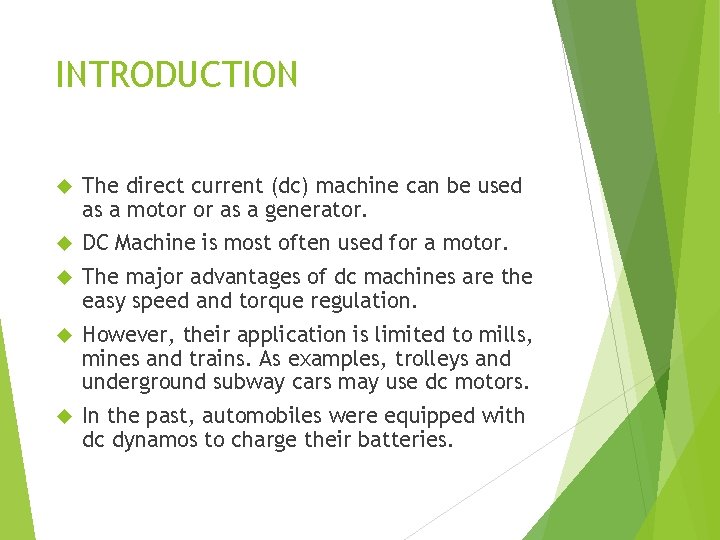
INTRODUCTION The direct current (dc) machine can be used as a motor or as a generator. DC Machine is most often used for a motor. The major advantages of dc machines are the easy speed and torque regulation. However, their application is limited to mills, mines and trains. As examples, trolleys and underground subway cars may use dc motors. In the past, automobiles were equipped with dc dynamos to charge their batteries.
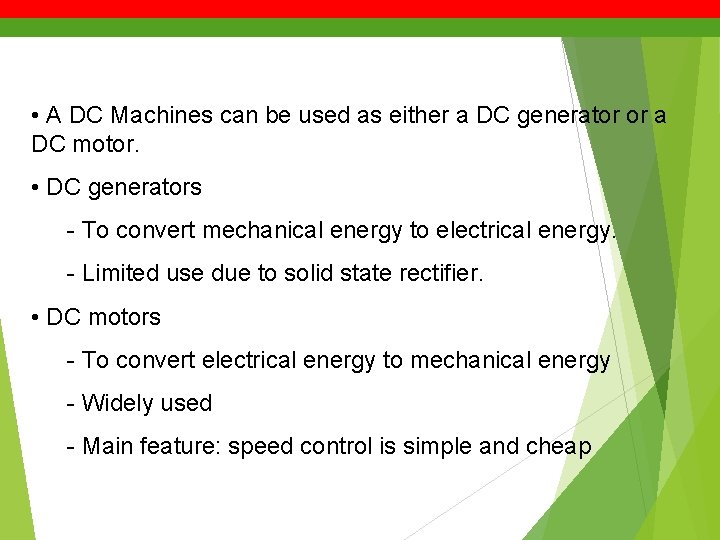
• A DC Machines can be used as either a DC generator or a DC motor. • DC generators - To convert mechanical energy to electrical energy. - Limited use due to solid state rectifier. • DC motors - To convert electrical energy to mechanical energy - Widely used - Main feature: speed control is simple and cheap
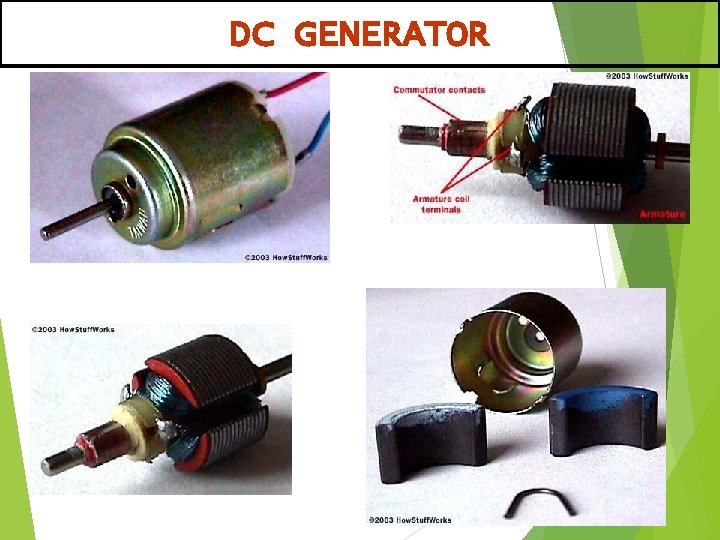
DC GENERATOR
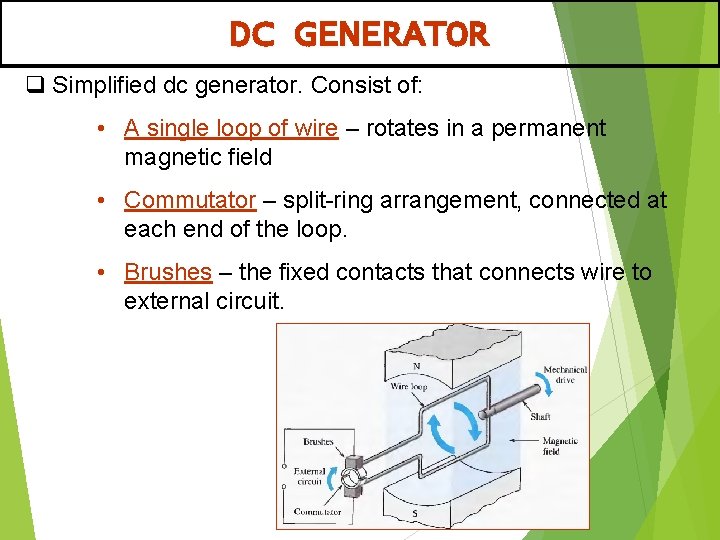
DC GENERATOR q Simplified dc generator. Consist of: • A single loop of wire – rotates in a permanent magnetic field • Commutator – split-ring arrangement, connected at each end of the loop. • Brushes – the fixed contacts that connects wire to external circuit.
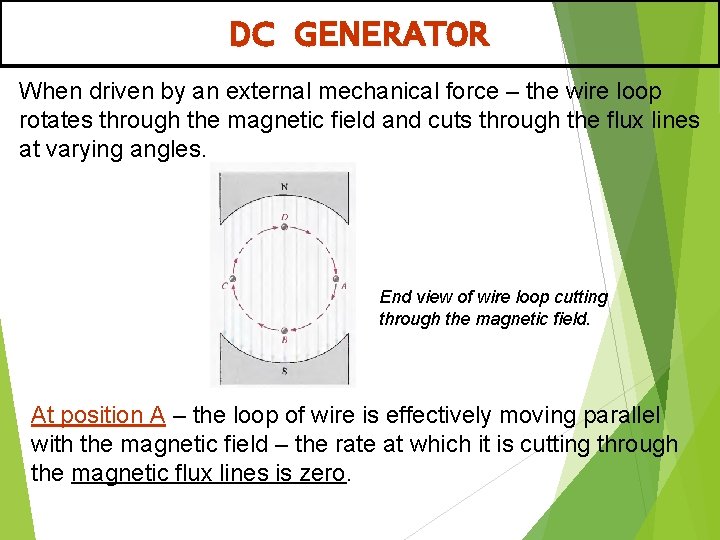
DC GENERATOR When driven by an external mechanical force – the wire loop rotates through the magnetic field and cuts through the flux lines at varying angles. End view of wire loop cutting through the magnetic field. At position A – the loop of wire is effectively moving parallel with the magnetic field – the rate at which it is cutting through the magnetic flux lines is zero.
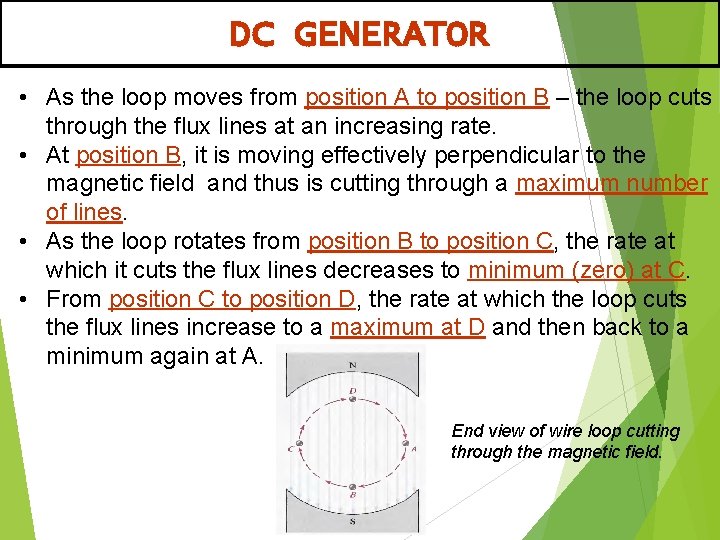
DC GENERATOR • As the loop moves from position A to position B – the loop cuts through the flux lines at an increasing rate. • At position B, it is moving effectively perpendicular to the magnetic field and thus is cutting through a maximum number of lines. • As the loop rotates from position B to position C, the rate at which it cuts the flux lines decreases to minimum (zero) at C. • From position C to position D, the rate at which the loop cuts the flux lines increase to a maximum at D and then back to a minimum again at A. End view of wire loop cutting through the magnetic field.
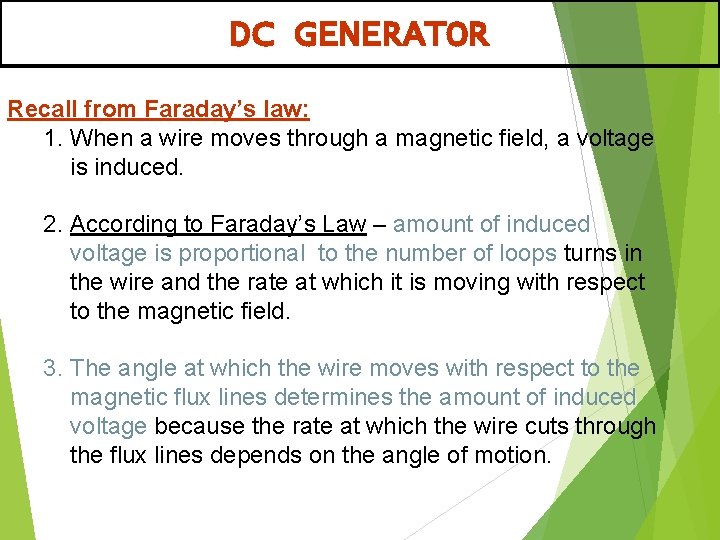
DC GENERATOR Recall from Faraday’s law: 1. When a wire moves through a magnetic field, a voltage is induced. 2. According to Faraday’s Law – amount of induced voltage is proportional to the number of loops turns in the wire and the rate at which it is moving with respect to the magnetic field. 3. The angle at which the wire moves with respect to the magnetic flux lines determines the amount of induced voltage because the rate at which the wire cuts through the flux lines depends on the angle of motion.
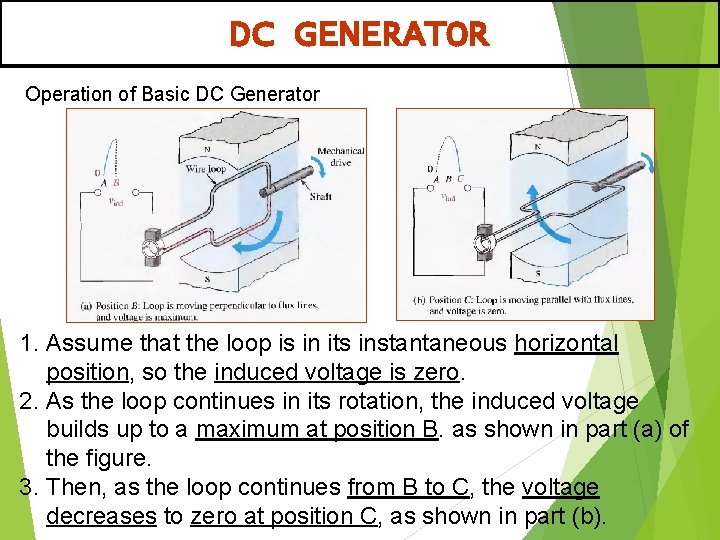
DC GENERATOR Operation of Basic DC Generator 1. Assume that the loop is in its instantaneous horizontal position, so the induced voltage is zero. 2. As the loop continues in its rotation, the induced voltage builds up to a maximum at position B. as shown in part (a) of the figure. 3. Then, as the loop continues from B to C, the voltage decreases to zero at position C, as shown in part (b).
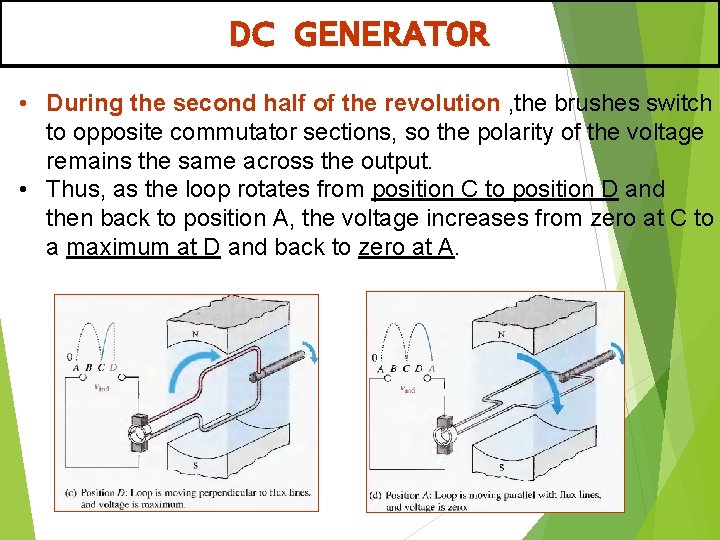
DC GENERATOR • During the second half of the revolution , the brushes switch to opposite commutator sections, so the polarity of the voltage remains the same across the output. • Thus, as the loop rotates from position C to position D and then back to position A, the voltage increases from zero at C to a maximum at D and back to zero at A.
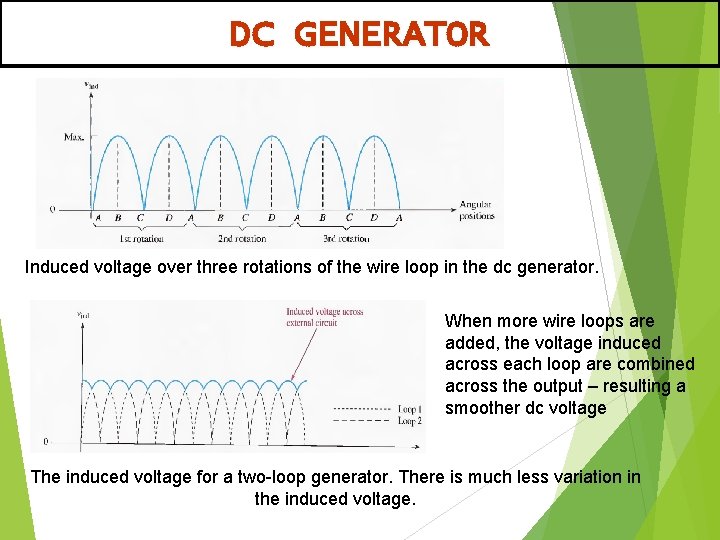
DC GENERATOR Induced voltage over three rotations of the wire loop in the dc generator. When more wire loops are added, the voltage induced across each loop are combined across the output – resulting a smoother dc voltage The induced voltage for a two-loop generator. There is much less variation in the induced voltage.
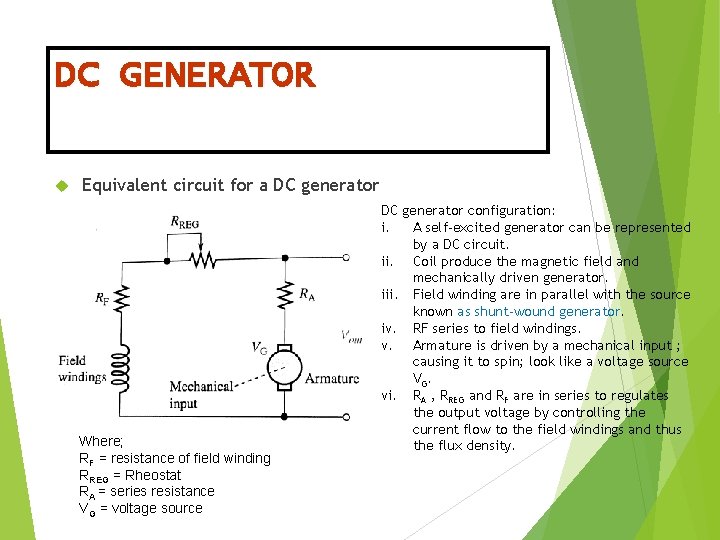
DC GENERATOR Equivalent circuit for a DC generator Where; RF = resistance of field winding RREG = Rheostat RA = series resistance VG = voltage source DC generator configuration: i. A self-excited generator can be represented by a DC circuit. ii. Coil produce the magnetic field and mechanically driven generator. iii. Field winding are in parallel with the source known as shunt-wound generator. iv. RF series to field windings. v. Armature is driven by a mechanical input ; causing it to spin; look like a voltage source V G. vi. RA , RREG and RF are in series to regulates the output voltage by controlling the current flow to the field windings and thus the flux density.
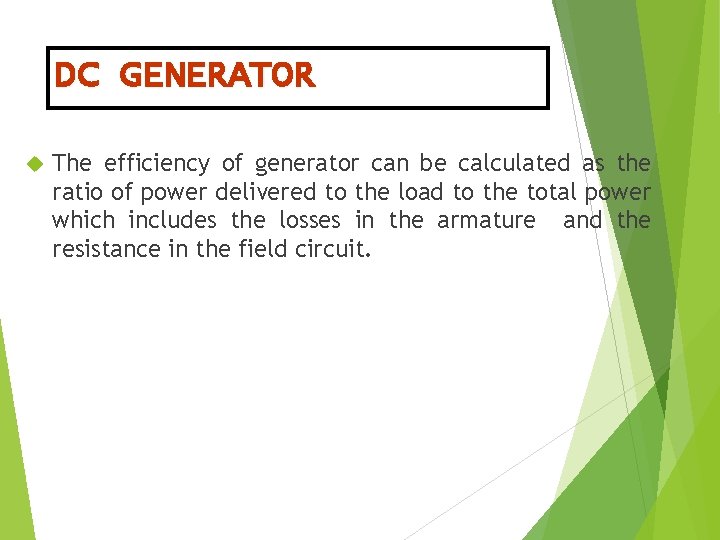
DC GENERATOR The efficiency of generator can be calculated as the ratio of power delivered to the load to the total power which includes the losses in the armature and the resistance in the field circuit.
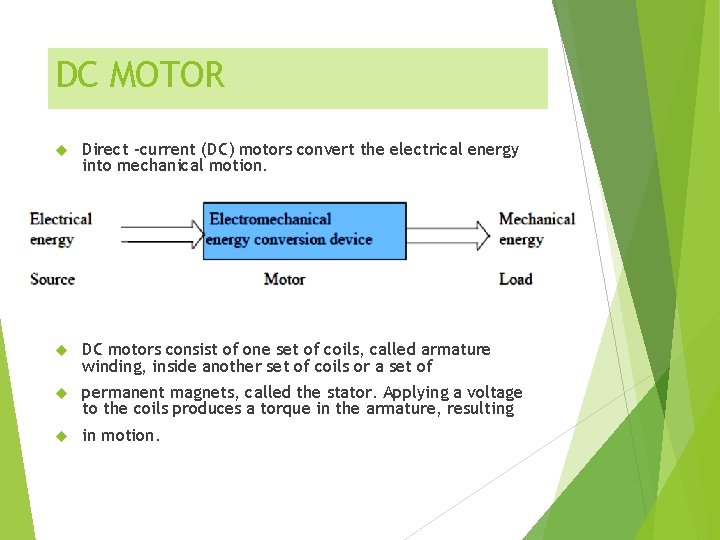
DC MOTOR Direct –current (DC) motors convert the electrical energy into mechanical motion. DC motors consist of one set of coils, called armature winding, inside another set of coils or a set of permanent magnets, called the stator. Applying a voltage to the coils produces a torque in the armature, resulting in motion.
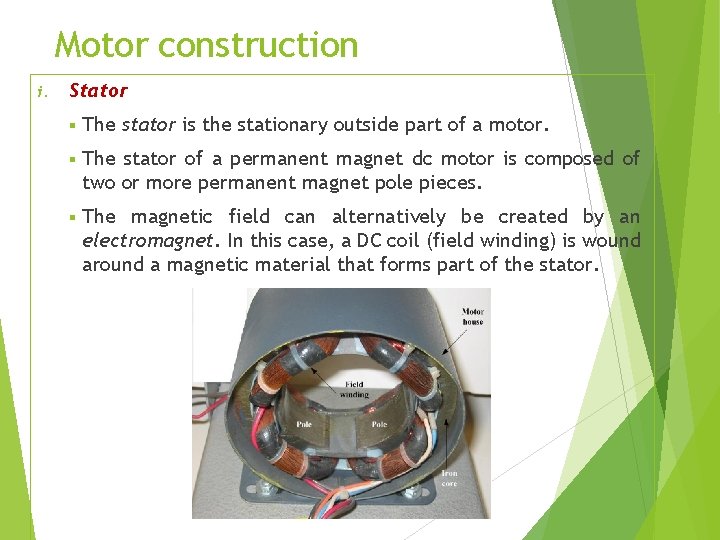
Motor construction i. Stator § The stator is the stationary outside part of a motor. § The stator of a permanent magnet dc motor is composed of two or more permanent magnet pole pieces. § The magnetic field can alternatively be created by an electromagnet. In this case, a DC coil (field winding) is wound around a magnetic material that forms part of the stator.
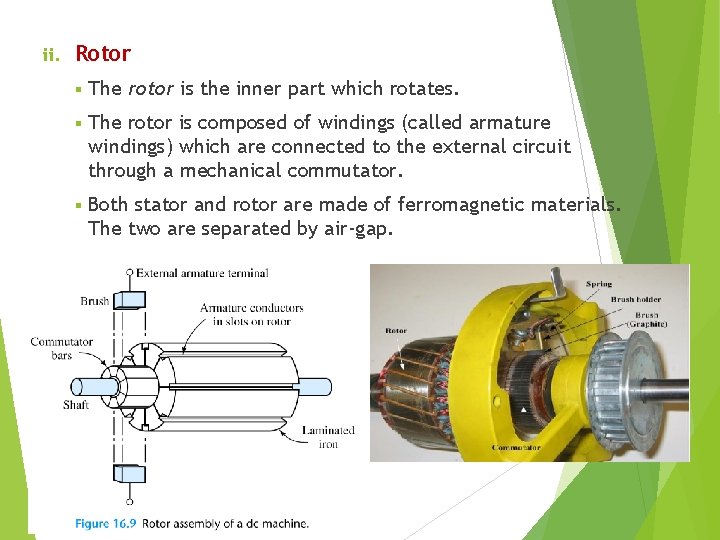
ii. Rotor § The rotor is the inner part which rotates. § The rotor is composed of windings (called armature windings) which are connected to the external circuit through a mechanical commutator. § Both stator and rotor are made of ferromagnetic materials. The two are separated by air-gap.
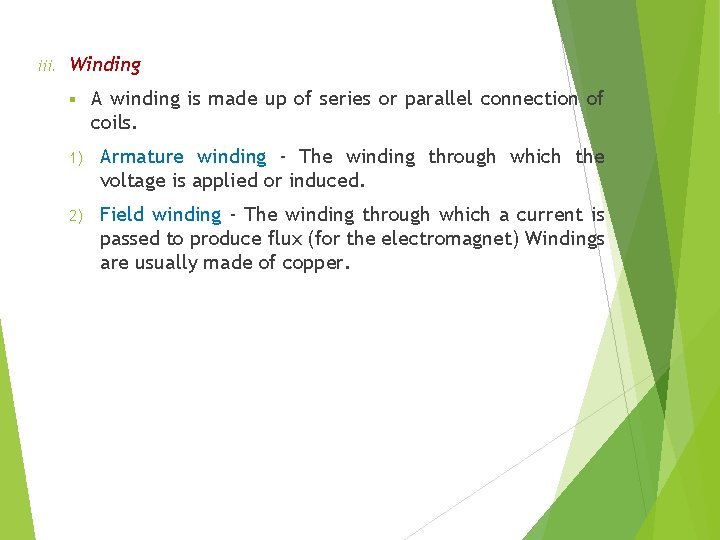
iii. Winding § A winding is made up of series or parallel connection of coils. 1) Armature winding - The winding through which the voltage is applied or induced. 2) Field winding - The winding through which a current is passed to produce flux (for the electromagnet) Windings are usually made of copper.
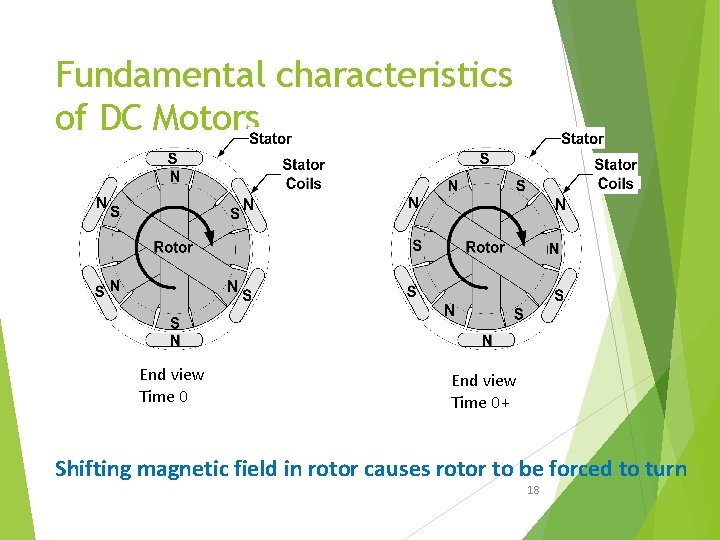
Fundamental characteristics of DC Motors End view Time 0+ Shifting magnetic field in rotor causes rotor to be forced to turn 18
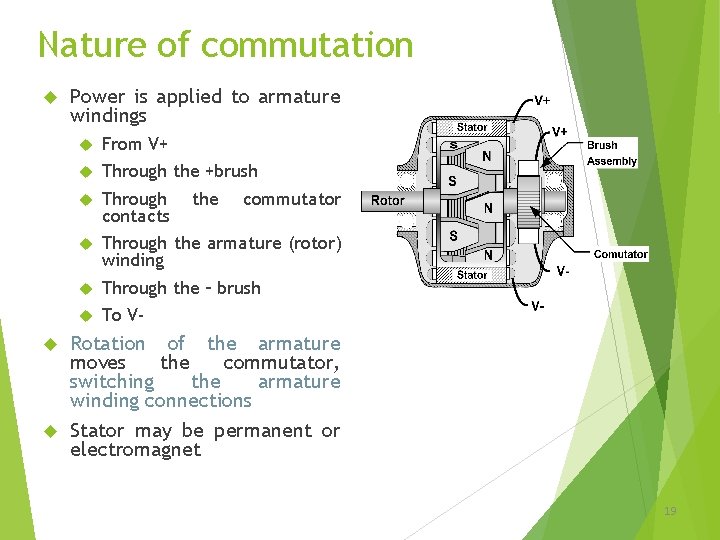
Nature of commutation Power is applied to armature windings From V+ Through the +brush Through contacts Through the armature (rotor) winding Through the – brush To V- the commutator Rotation of the armature moves the commutator, switching the armature winding connections Stator may be permanent or electromagnet 19
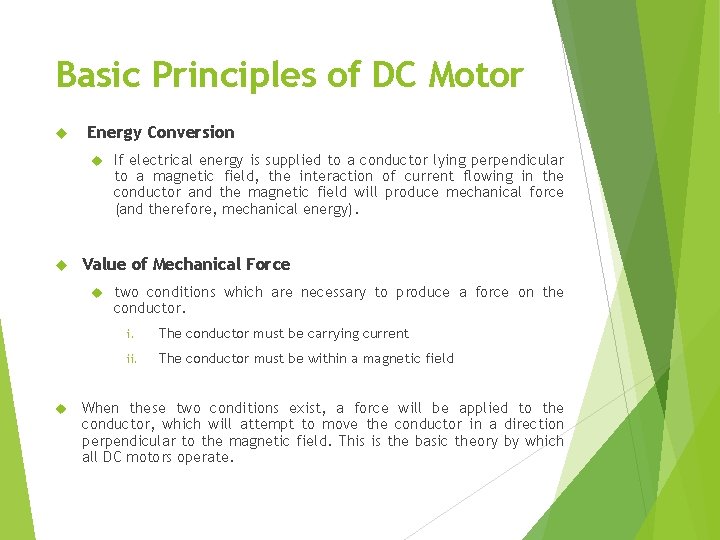
Basic Principles of DC Motor Energy Conversion Value of Mechanical Force If electrical energy is supplied to a conductor lying perpendicular to a magnetic field, the interaction of current flowing in the conductor and the magnetic field will produce mechanical force (and therefore, mechanical energy). two conditions which are necessary to produce a force on the conductor. i. The conductor must be carrying current ii. The conductor must be within a magnetic field When these two conditions exist, a force will be applied to the conductor, which will attempt to move the conductor in a direction perpendicular to the magnetic field. This is the basic theory by which all DC motors operate.
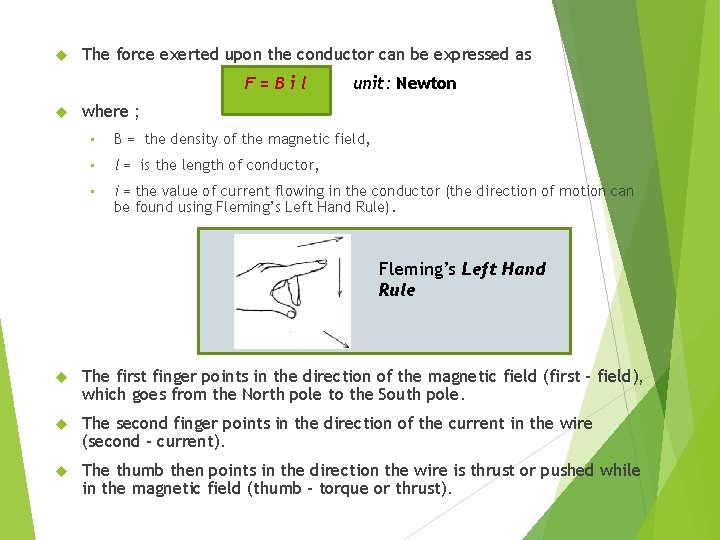
The force exerted upon the conductor can be expressed as F=Bil unit: Newton where ; • B = the density of the magnetic field, • l = is the length of conductor, • i = the value of current flowing in the conductor (the direction of motion can be found using Fleming’s Left Hand Rule). Fleming’s Left Hand Rule The first finger points in the direction of the magnetic field (first - field), which goes from the North pole to the South pole. The second finger points in the direction of the current in the wire (second - current). The thumb then points in the direction the wire is thrust or pushed while in the magnetic field (thumb - torque or thrust).
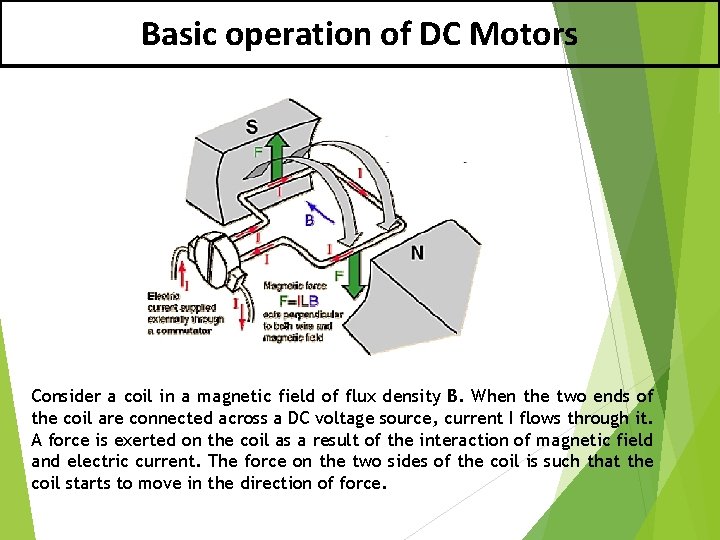
Basic operation of DC Motors Consider a coil in a magnetic field of flux density B. When the two ends of the coil are connected across a DC voltage source, current I flows through it. A force is exerted on the coil as a result of the interaction of magnetic field and electric current. The force on the two sides of the coil is such that the coil starts to move in the direction of force.
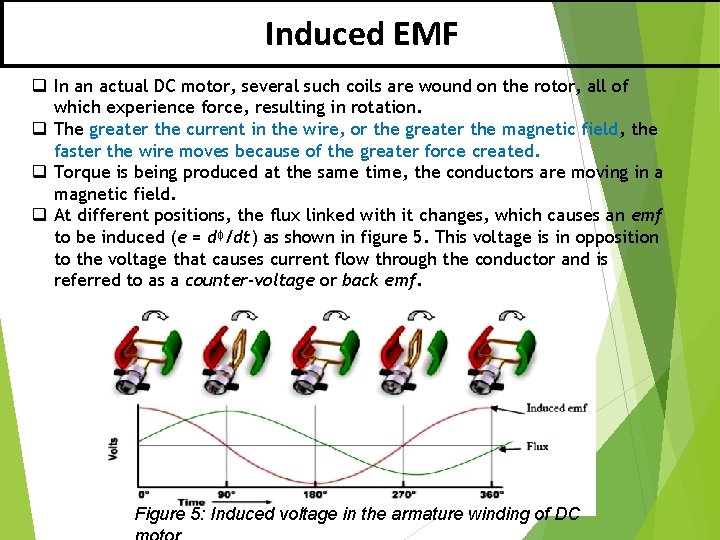
Induced EMF q In an actual DC motor, several such coils are wound on the rotor, all of which experience force, resulting in rotation. q The greater the current in the wire, or the greater the magnetic field, the faster the wire moves because of the greater force created. q Torque is being produced at the same time, the conductors are moving in a magnetic field. q At different positions, the flux linked with it changes, which causes an emf to be induced (e = dᶲ/dt) as shown in figure 5. This voltage is in opposition to the voltage that causes current flow through the conductor and is referred to as a counter-voltage or back emf. Figure 5: Induced voltage in the armature winding of DC
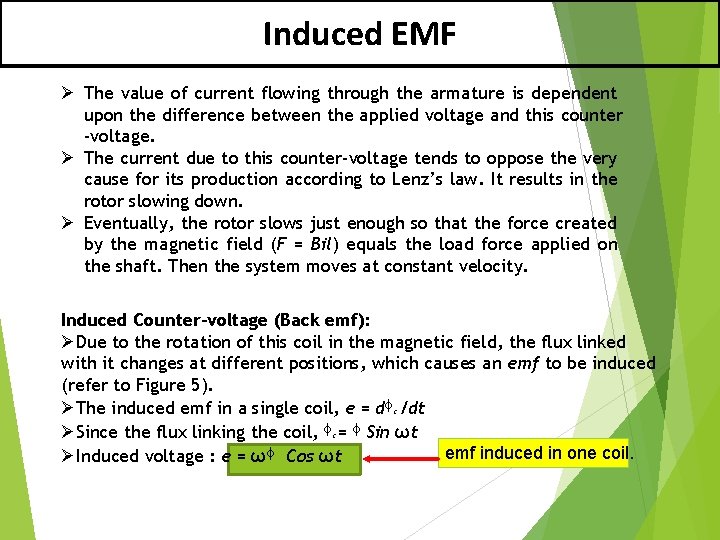
Induced EMF Ø The value of current flowing through the armature is dependent upon the difference between the applied voltage and this counter -voltage. Ø The current due to this counter-voltage tends to oppose the very cause for its production according to Lenz’s law. It results in the rotor slowing down. Ø Eventually, the rotor slows just enough so that the force created by the magnetic field (F = Bil) equals the load force applied on the shaft. Then the system moves at constant velocity. Induced Counter-voltage (Back emf): ØDue to the rotation of this coil in the magnetic field, the flux linked with it changes at different positions, which causes an emf to be induced (refer to Figure 5). ØThe induced emf in a single coil, e = dᶲc /dt ØSince the flux linking the coil, ᶲc = ᶲ Sin ωt emf induced in one coil. ØInduced voltage : e = ωᶲ Cos ωt
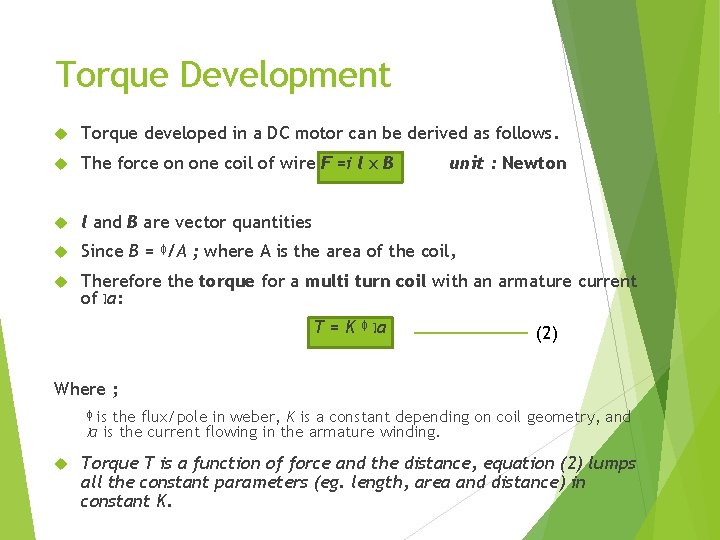
Torque Development Torque developed in a DC motor can be derived as follows. The force on one coil of wire F =i l x B l and B are vector quantities Since B = ᶲ/A ; where A is the area of the coil, Therefore the torque for a multi turn coil with an armature current of Ia: T = K ᶲ Ia unit : Newton (2) Where ; ᶲ is the flux/pole in weber, K is a constant depending on coil geometry, and Ia is the current flowing in the armature winding. Torque T is a function of force and the distance, equation (2) lumps all the constant parameters (eg. length, area and distance) in constant K.
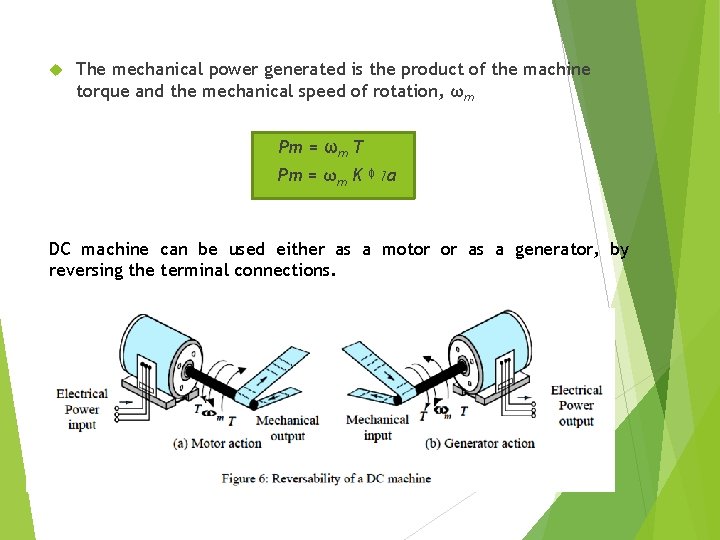
The mechanical power generated is the product of the machine torque and the mechanical speed of rotation, ωm Pm = ωm T Pm = ωm K ᶲ I a DC machine can be used either as a motor or as a generator, by reversing the terminal connections.
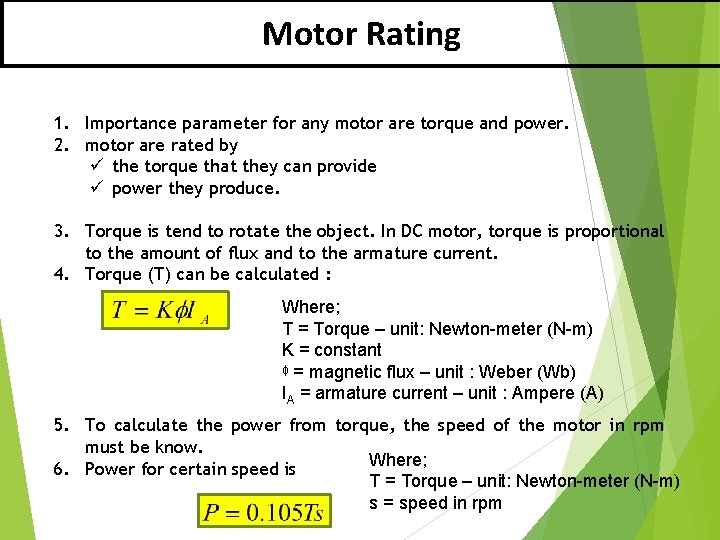
Motor Rating 1. Importance parameter for any motor are torque and power. 2. motor are rated by ü the torque that they can provide ü power they produce. 3. Torque is tend to rotate the object. In DC motor, torque is proportional to the amount of flux and to the armature current. 4. Torque (T) can be calculated : Where; T = Torque – unit: Newton-meter (N-m) K = constant ᶲ = magnetic flux – unit : Weber (Wb) IA = armature current – unit : Ampere (A) 5. To calculate the power from torque, the speed of the motor in rpm must be know. Where; 6. Power for certain speed is T = Torque – unit: Newton-meter (N-m) s = speed in rpm
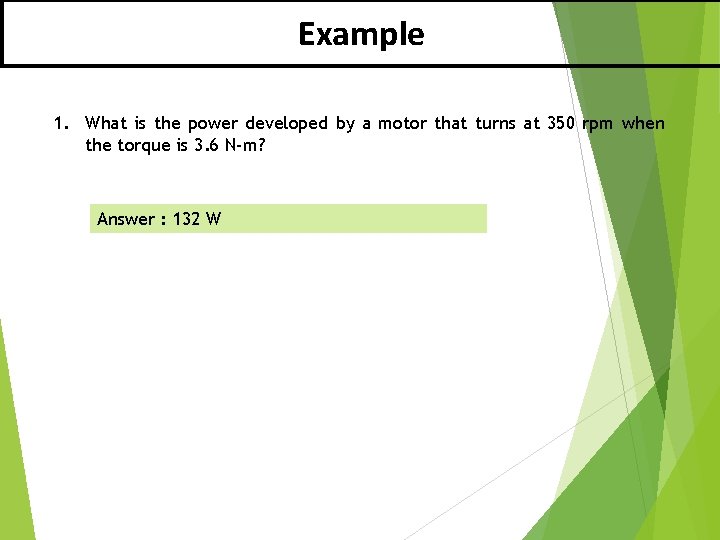
Example 1. What is the power developed by a motor that turns at 350 rpm when the torque is 3. 6 N-m? Answer : 132 W
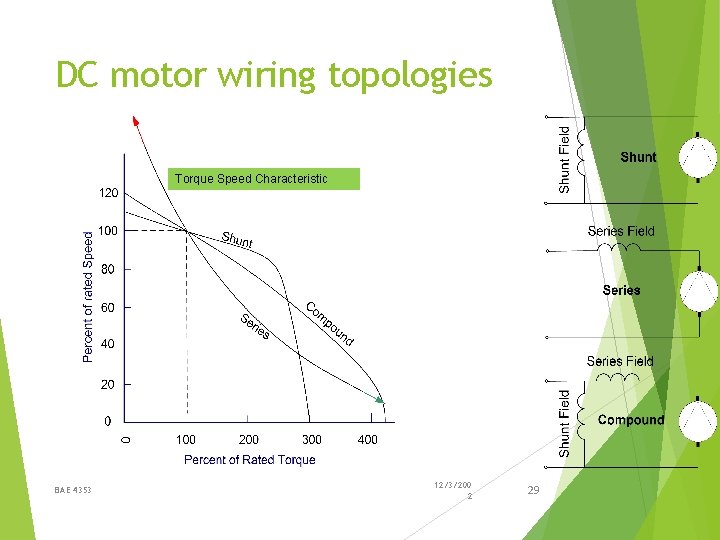
DC motor wiring topologies Torque Speed Characteristic BAE 4353 12/3/200 2 29
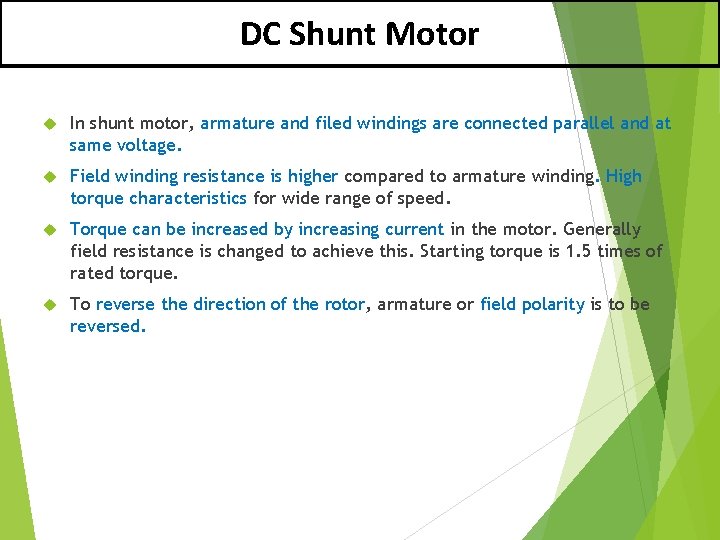
DC Shunt Motor In shunt motor, armature and filed windings are connected parallel and at same voltage. Field winding resistance is higher compared to armature winding. High torque characteristics for wide range of speed. Torque can be increased by increasing current in the motor. Generally field resistance is changed to achieve this. Starting torque is 1. 5 times of rated torque. To reverse the direction of the rotor, armature or field polarity is to be reversed.
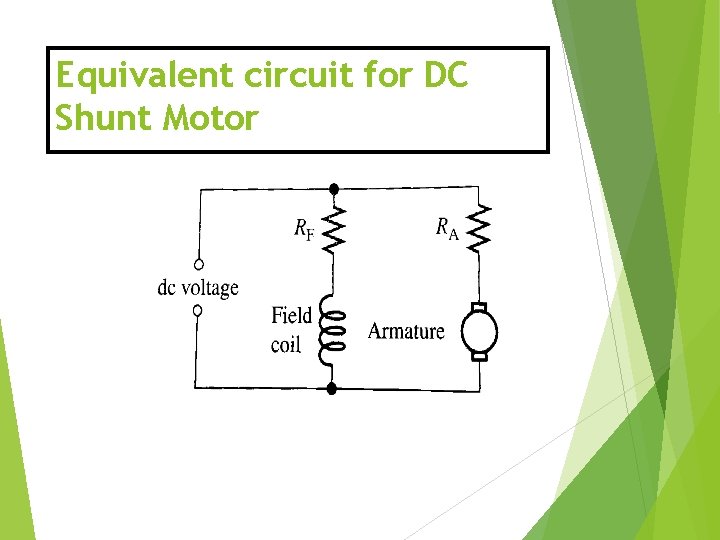
Equivalent circuit for DC Shunt Motor
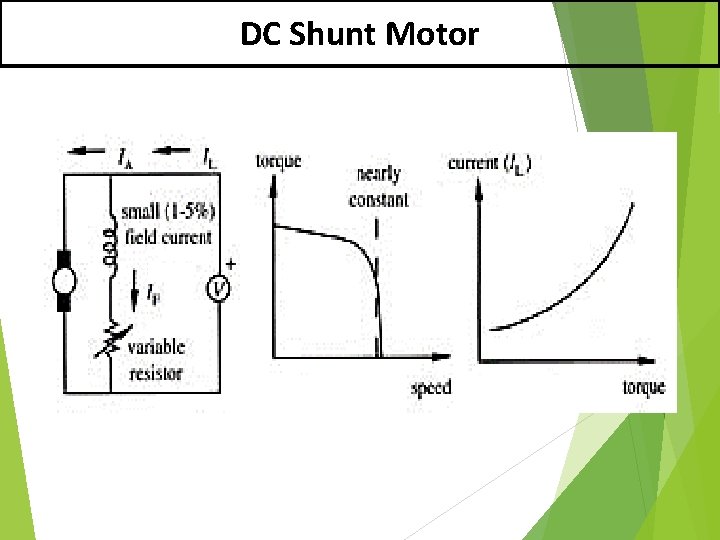
DC Shunt Motor
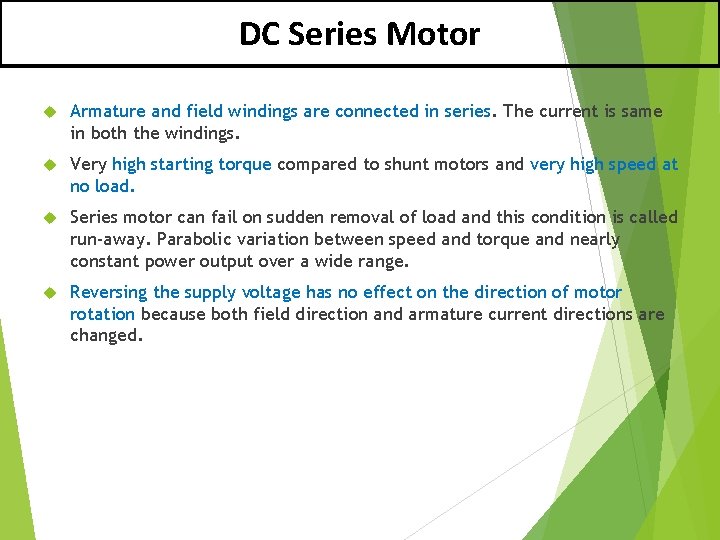
DC Series Motor Armature and field windings are connected in series. The current is same in both the windings. Very high starting torque compared to shunt motors and very high speed at no load. Series motor can fail on sudden removal of load and this condition is called run-away. Parabolic variation between speed and torque and nearly constant power output over a wide range. Reversing the supply voltage has no effect on the direction of motor rotation because both field direction and armature current directions are changed.
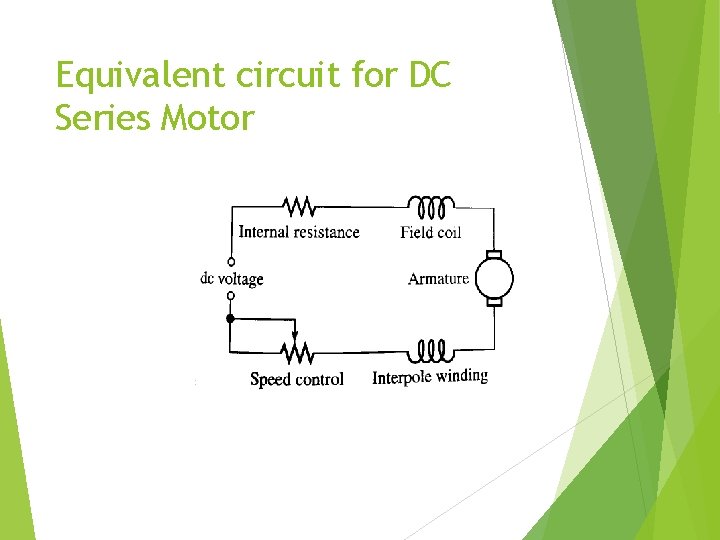
Equivalent circuit for DC Series Motor
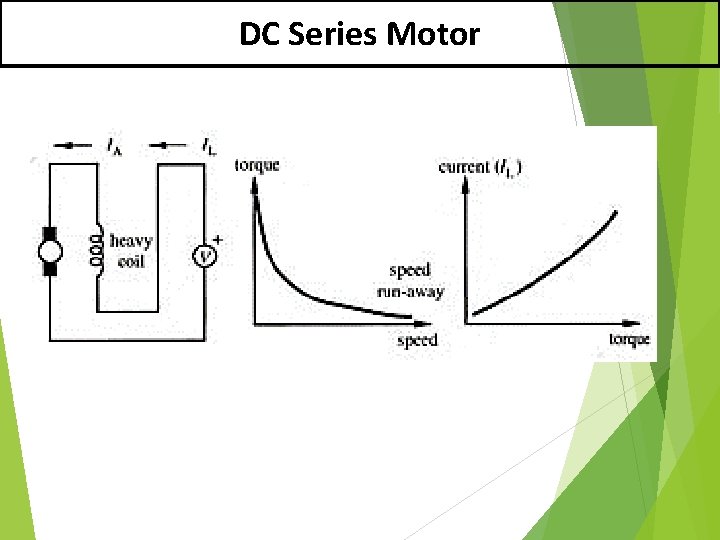
DC Series Motor
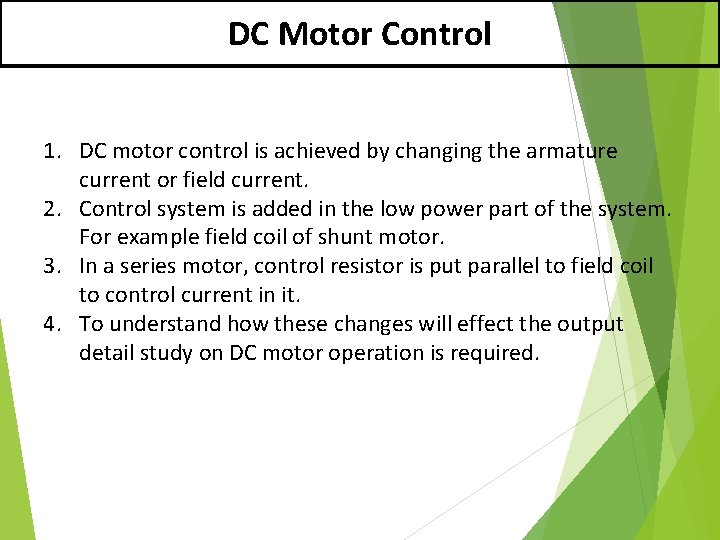
DC Motor Control 1. DC motor control is achieved by changing the armature current or field current. 2. Control system is added in the low power part of the system. For example field coil of shunt motor. 3. In a series motor, control resistor is put parallel to field coil to control current in it. 4. To understand how these changes will effect the output detail study on DC motor operation is required.
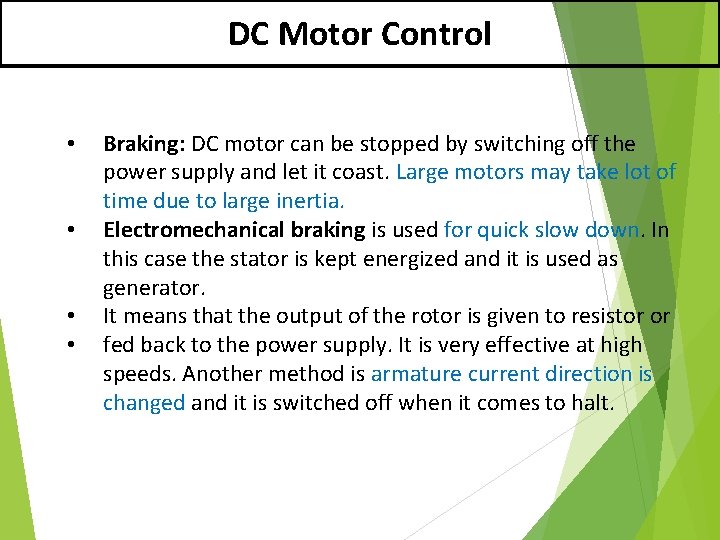
DC Motor Control • • Braking: DC motor can be stopped by switching off the power supply and let it coast. Large motors may take lot of time due to large inertia. Electromechanical braking is used for quick slow down. In this case the stator is kept energized and it is used as generator. It means that the output of the rotor is given to resistor or fed back to the power supply. It is very effective at high speeds. Another method is armature current direction is changed and it is switched off when it comes to halt.
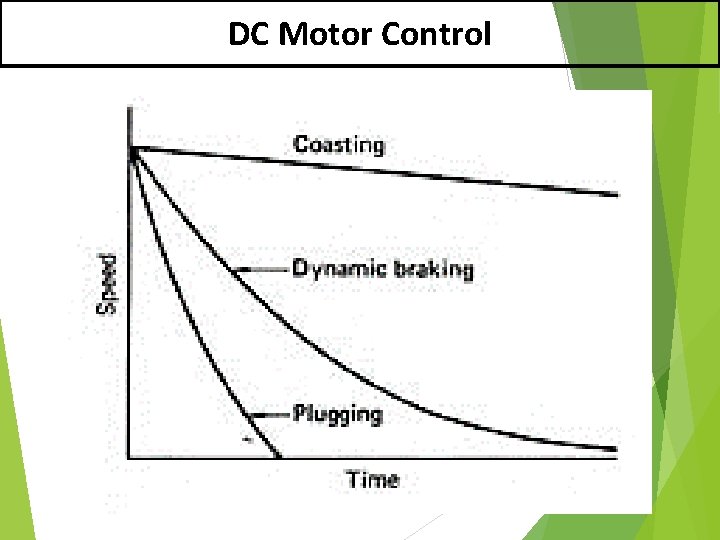
DC Motor Control
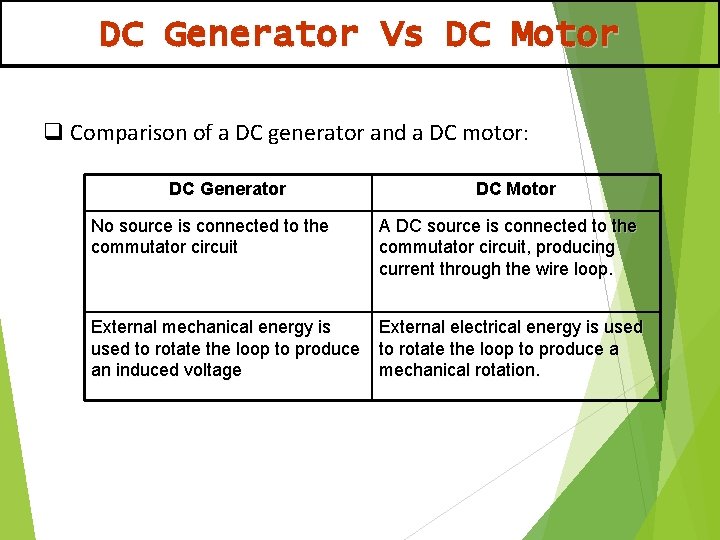
DC Generator Vs DC Motor q Comparison of a DC generator and a DC motor: DC Generator DC Motor No source is connected to the commutator circuit A DC source is connected to the commutator circuit, producing current through the wire loop. External mechanical energy is used to rotate the loop to produce an induced voltage External electrical energy is used to rotate the loop to produce a mechanical rotation.
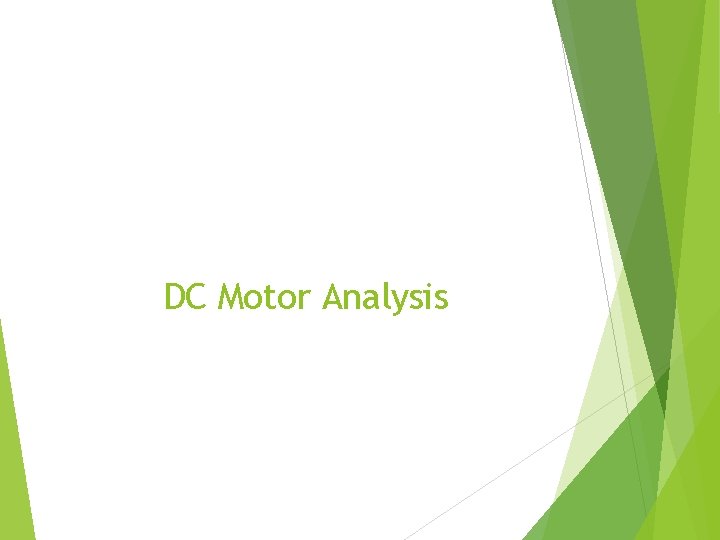
DC Motor Analysis
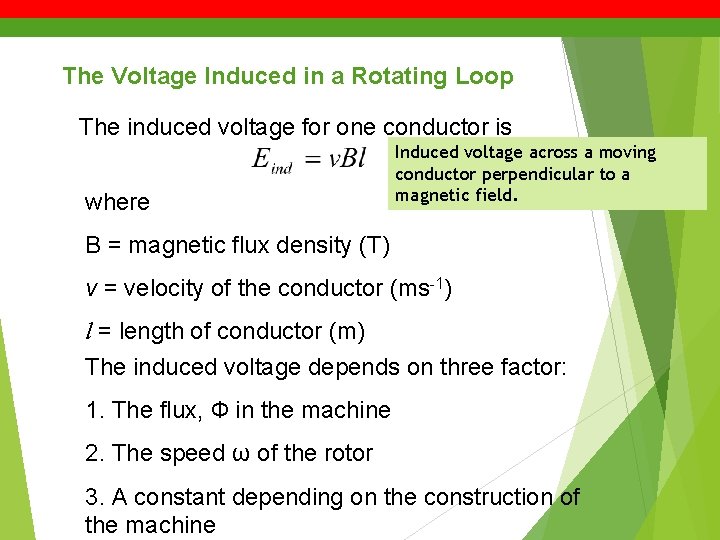
The Voltage Induced in a Rotating Loop The induced voltage for one conductor is where Induced voltage across a moving conductor perpendicular to a magnetic field. B = magnetic flux density (T) v = velocity of the conductor (ms-1) l = length of conductor (m) The induced voltage depends on three factor: 1. The flux, Ф in the machine 2. The speed ω of the rotor 3. A constant depending on the construction of the machine
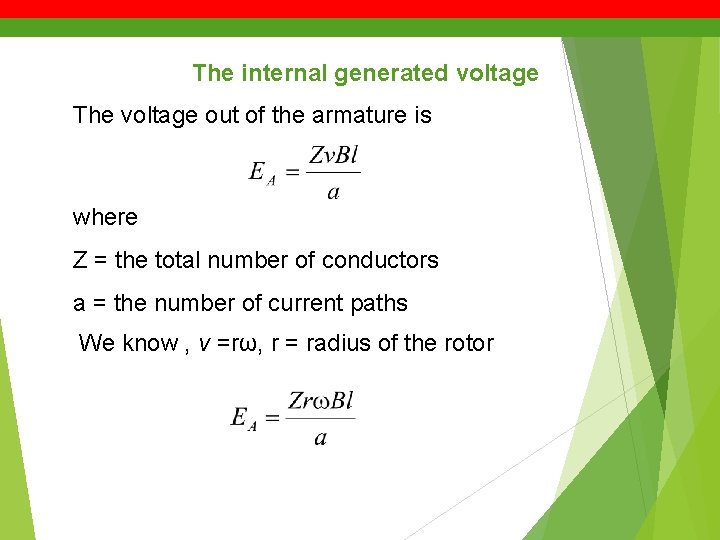
The internal generated voltage The voltage out of the armature is where Z = the total number of conductors a = the number of current paths We know , v =rω, r = radius of the rotor
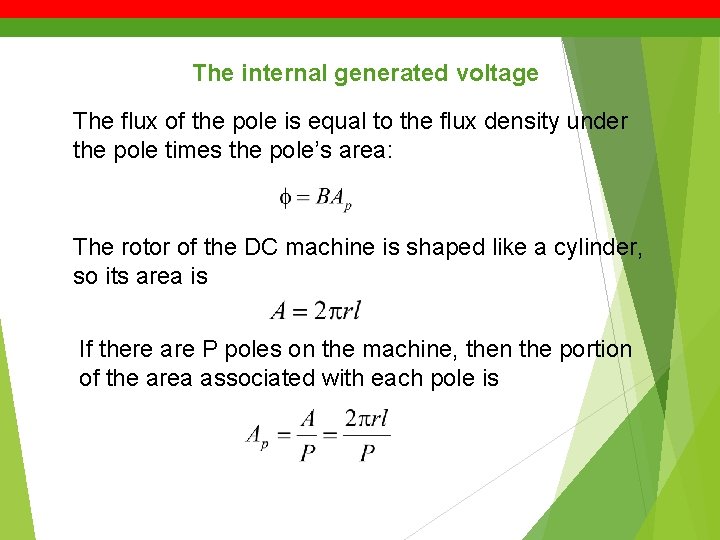
The internal generated voltage The flux of the pole is equal to the flux density under the pole times the pole’s area: The rotor of the DC machine is shaped like a cylinder, so its area is If there are P poles on the machine, then the portion of the area associated with each pole is
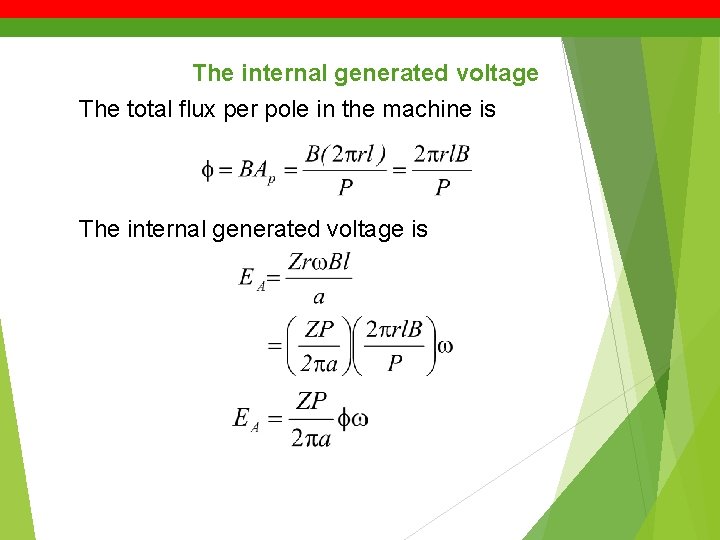
The internal generated voltage The total flux per pole in the machine is The internal generated voltage is
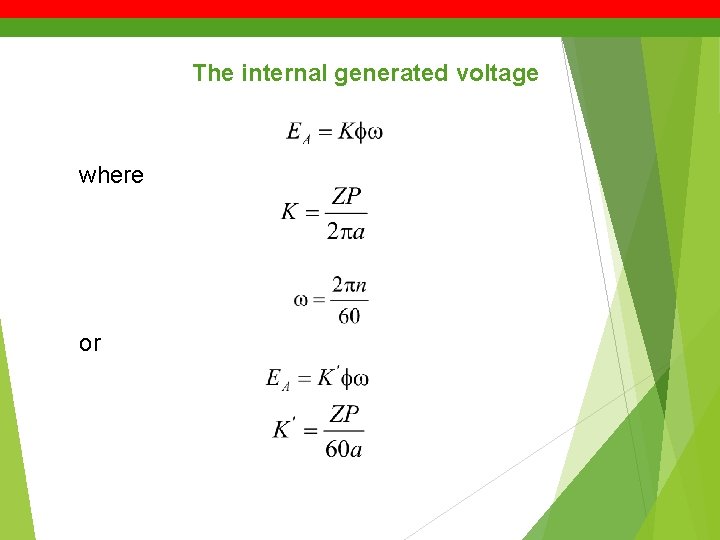
The internal generated voltage where or
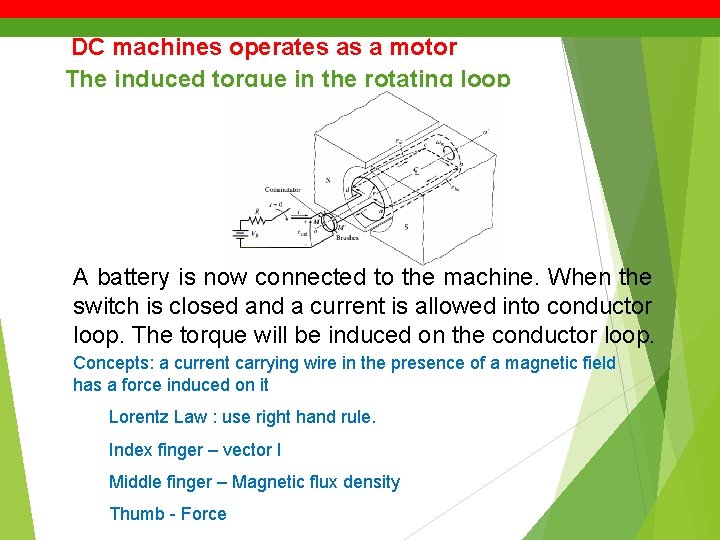
DC machines operates as a motor The induced torque in the rotating loop A battery is now connected to the machine. When the switch is closed and a current is allowed into conductor loop. The torque will be induced on the conductor loop. Concepts: a current carrying wire in the presence of a magnetic field has a force induced on it Lorentz Law : use right hand rule. Index finger – vector l Middle finger – Magnetic flux density Thumb - Force
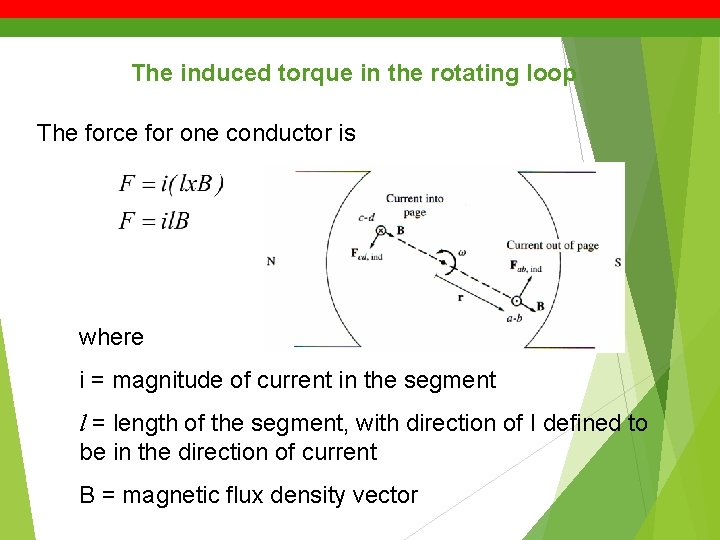
The induced torque in the rotating loop The force for one conductor is where i = magnitude of current in the segment l = length of the segment, with direction of I defined to be in the direction of current B = magnetic flux density vector
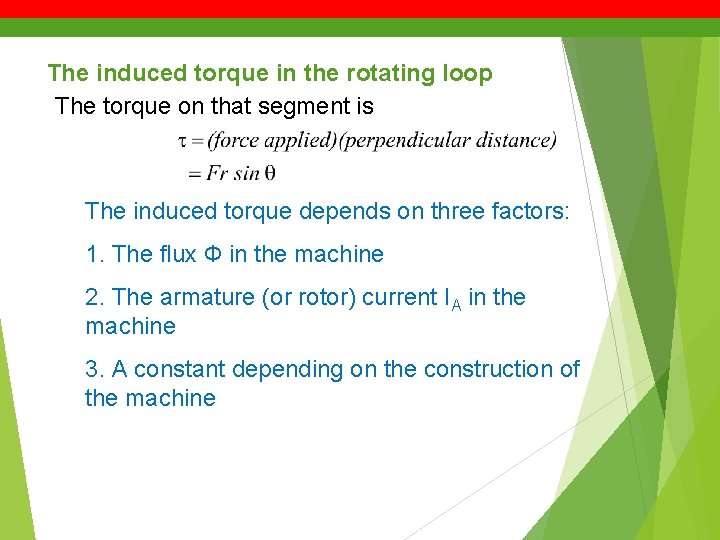
The induced torque in the rotating loop The torque on that segment is The induced torque depends on three factors: 1. The flux Ф in the machine 2. The armature (or rotor) current IA in the machine 3. A constant depending on the construction of the machine
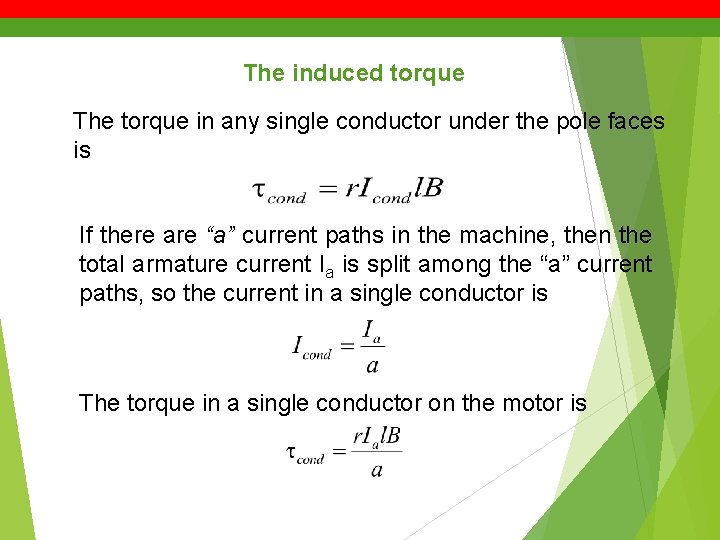
The induced torque The torque in any single conductor under the pole faces is If there are “a” current paths in the machine, then the total armature current Ia is split among the “a” current paths, so the current in a single conductor is The torque in a single conductor on the motor is
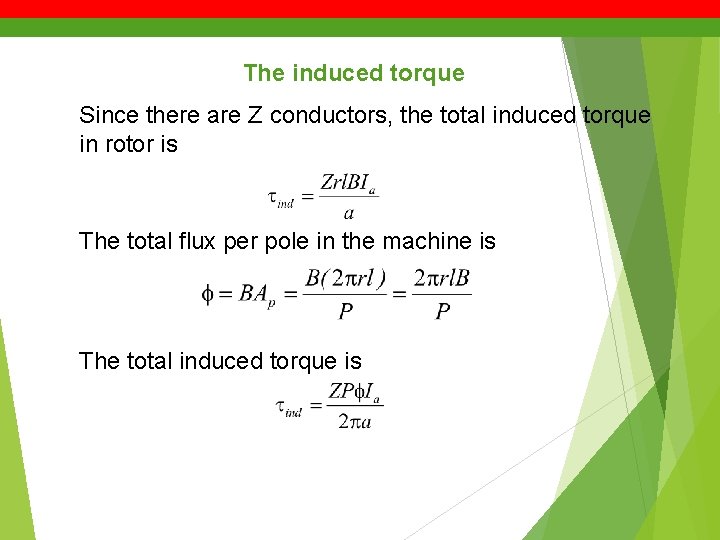
The induced torque Since there are Z conductors, the total induced torque in rotor is The total flux per pole in the machine is The total induced torque is
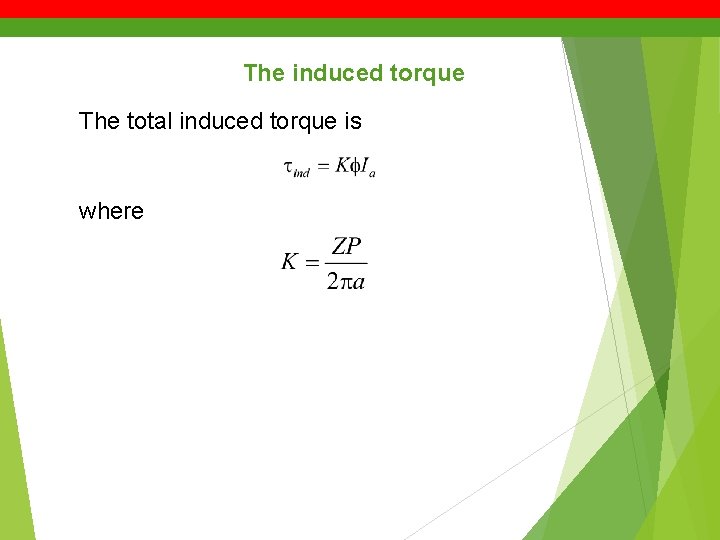
The induced torque The total induced torque is where
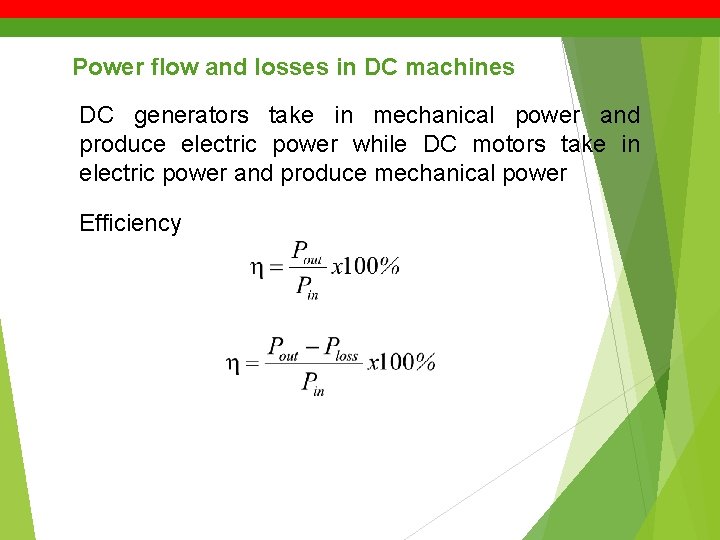
Power flow and losses in DC machines DC generators take in mechanical power and produce electric power while DC motors take in electric power and produce mechanical power Efficiency
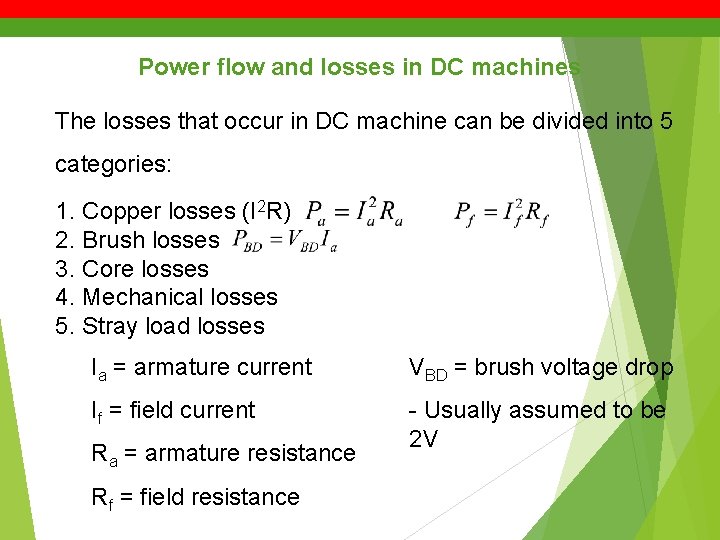
Power flow and losses in DC machines The losses that occur in DC machine can be divided into 5 categories: 1. Copper losses (I 2 R) 2. Brush losses 3. Core losses 4. Mechanical losses 5. Stray load losses Ia = armature current VBD = brush voltage drop If = field current - Usually assumed to be 2 V Ra = armature resistance Rf = field resistance
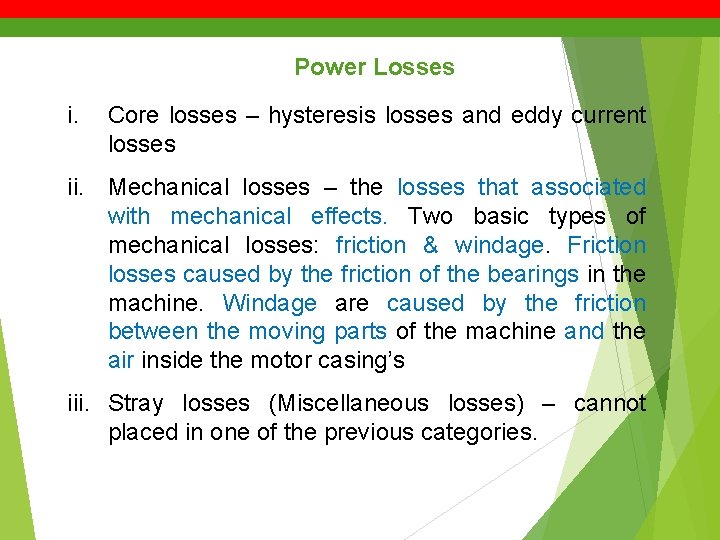
Power Losses i. Core losses – hysteresis losses and eddy current losses ii. Mechanical losses – the losses that associated with mechanical effects. Two basic types of mechanical losses: friction & windage. Friction losses caused by the friction of the bearings in the machine. Windage are caused by the friction between the moving parts of the machine and the air inside the motor casing’s iii. Stray losses (Miscellaneous losses) – cannot placed in one of the previous categories.
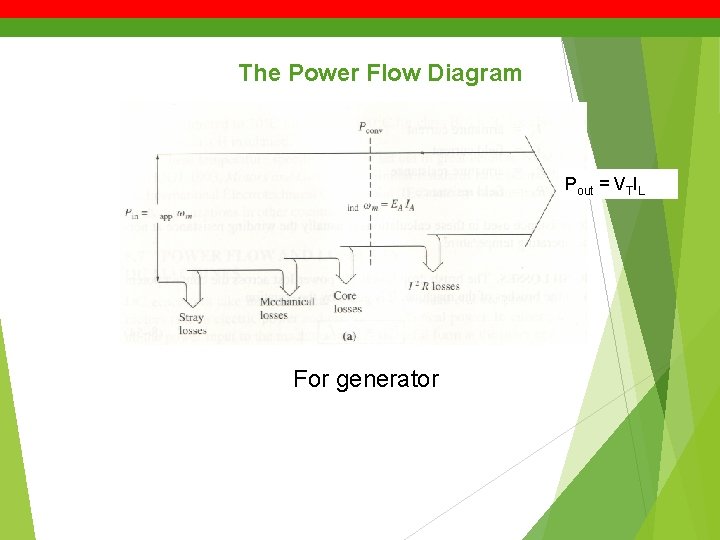
The Power Flow Diagram Pout = VTIL For generator
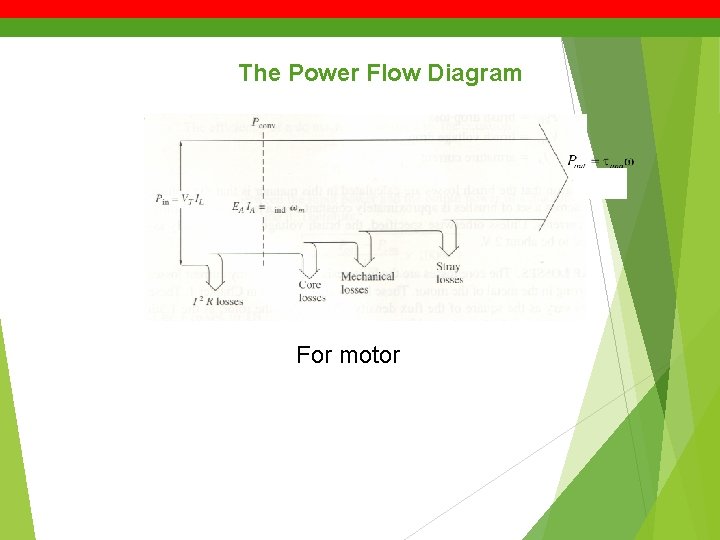
The Power Flow Diagram For motor
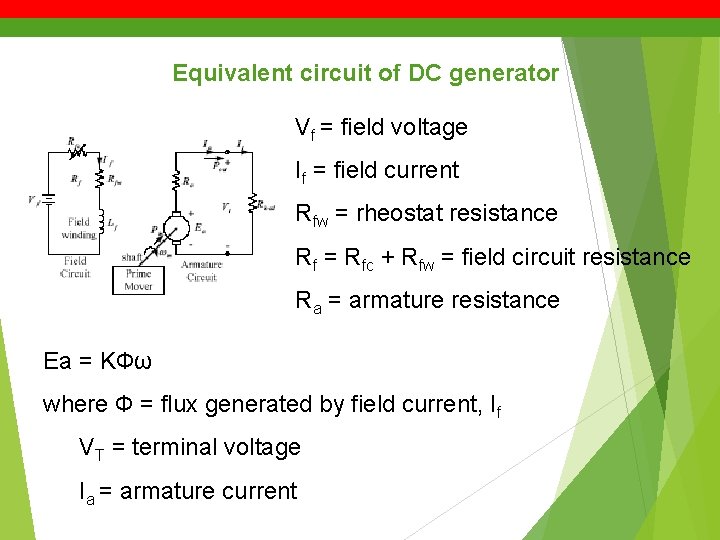
Equivalent circuit of DC generator Vf = field voltage If = field current Rfw = rheostat resistance Rf = Rfc + Rfw = field circuit resistance Ra = armature resistance Ea = KФω where Ф = flux generated by field current, If VT = terminal voltage Ia = armature current
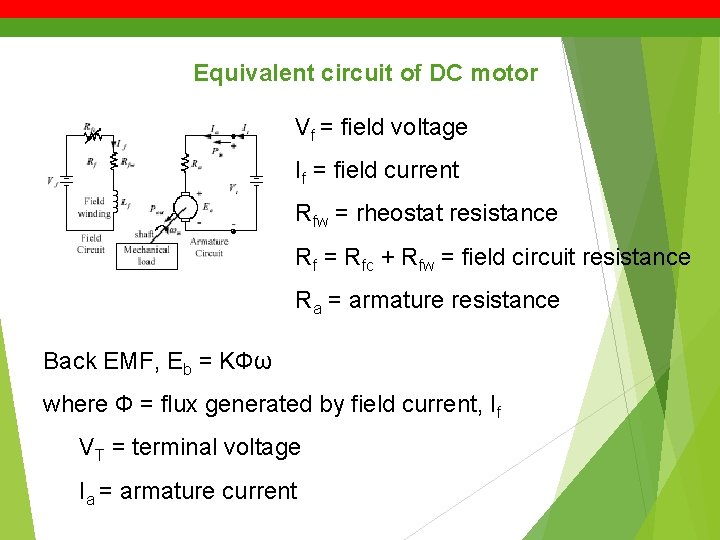
Equivalent circuit of DC motor Vf = field voltage If = field current Rfw = rheostat resistance Rf = Rfc + Rfw = field circuit resistance Ra = armature resistance Back EMF, Eb = KФω where Ф = flux generated by field current, If VT = terminal voltage Ia = armature current
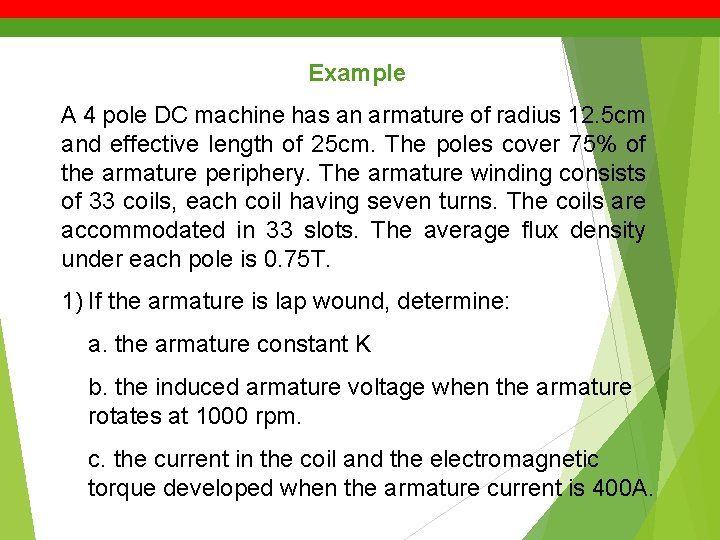
Example A 4 pole DC machine has an armature of radius 12. 5 cm and effective length of 25 cm. The poles cover 75% of the armature periphery. The armature winding consists of 33 coils, each coil having seven turns. The coils are accommodated in 33 slots. The average flux density under each pole is 0. 75 T. 1) If the armature is lap wound, determine: a. the armature constant K b. the induced armature voltage when the armature rotates at 1000 rpm. c. the current in the coil and the electromagnetic torque developed when the armature current is 400 A.
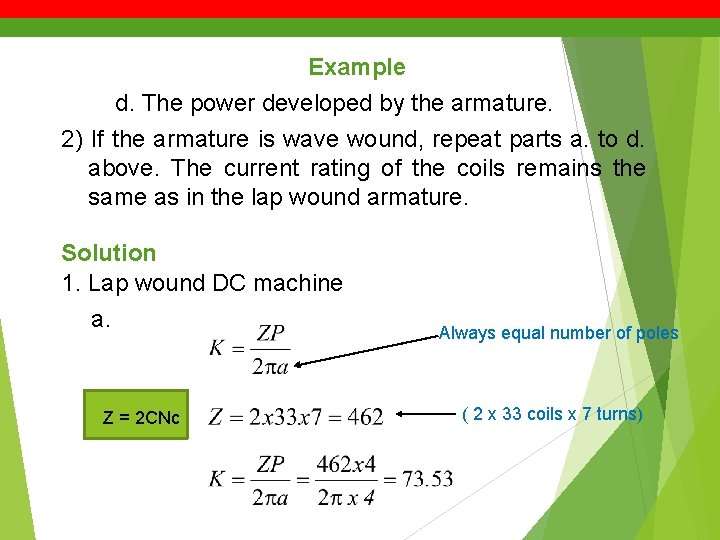
Example d. The power developed by the armature. 2) If the armature is wave wound, repeat parts a. to d. above. The current rating of the coils remains the same as in the lap wound armature. Solution 1. Lap wound DC machine a. Z = 2 CNc Always equal number of poles ( 2 x 33 coils x 7 turns)
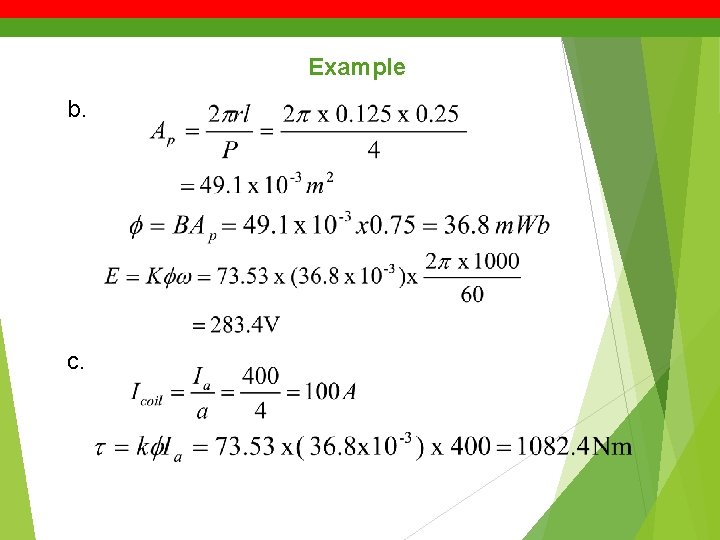
Example b. c.
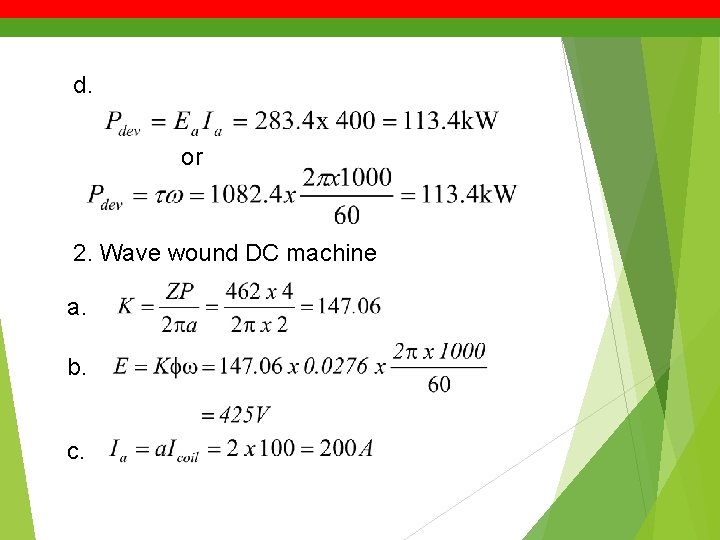
d. or 2. Wave wound DC machine a. b. c.
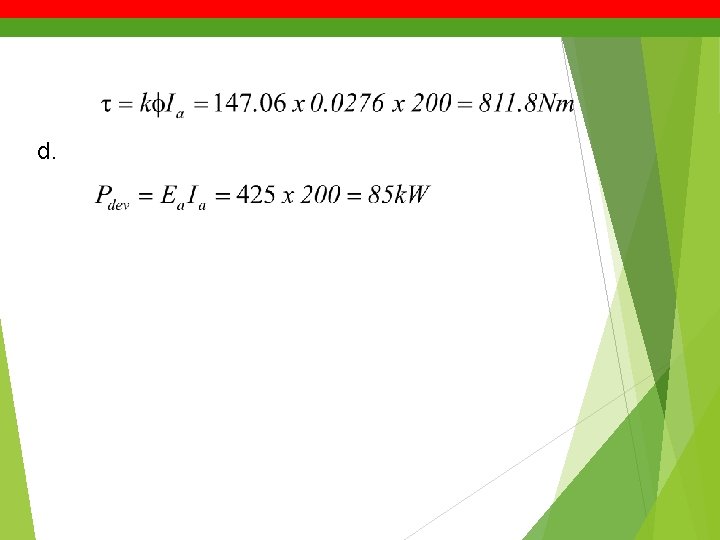
d.
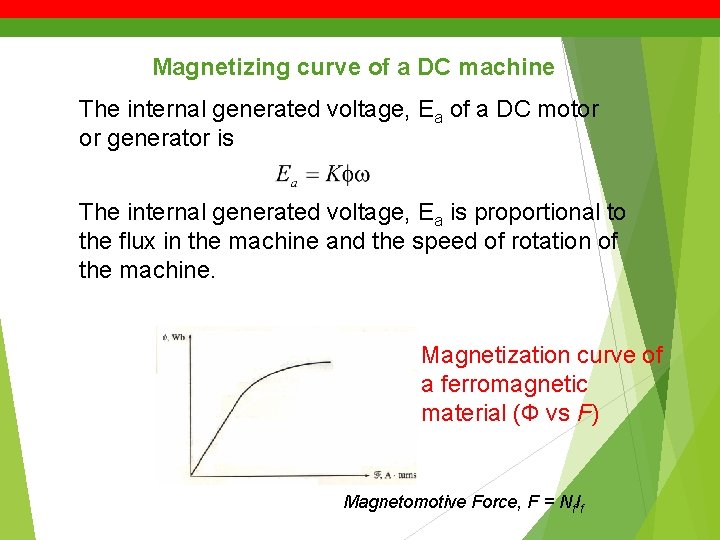
Magnetizing curve of a DC machine The internal generated voltage, Ea of a DC motor or generator is The internal generated voltage, Ea is proportional to the flux in the machine and the speed of rotation of the machine. Magnetization curve of a ferromagnetic material (Ф vs F) Magnetomotive Force, F = Nf. If
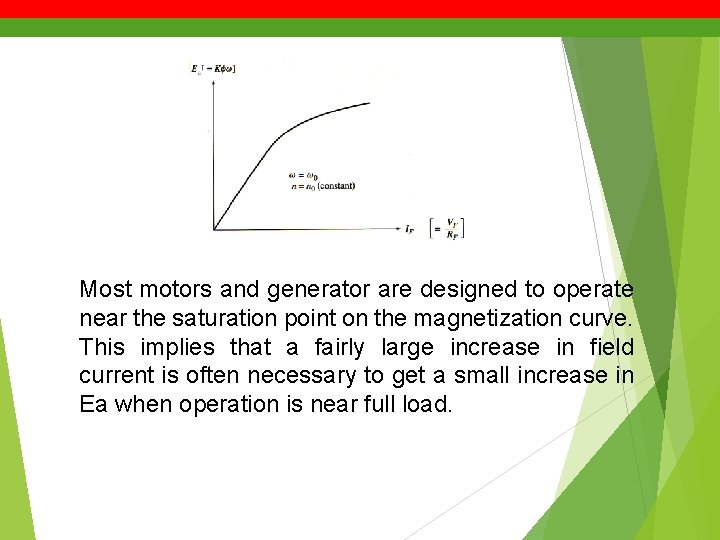
Most motors and generator are designed to operate near the saturation point on the magnetization curve. This implies that a fairly large increase in field current is often necessary to get a small increase in Ea when operation is near full load.
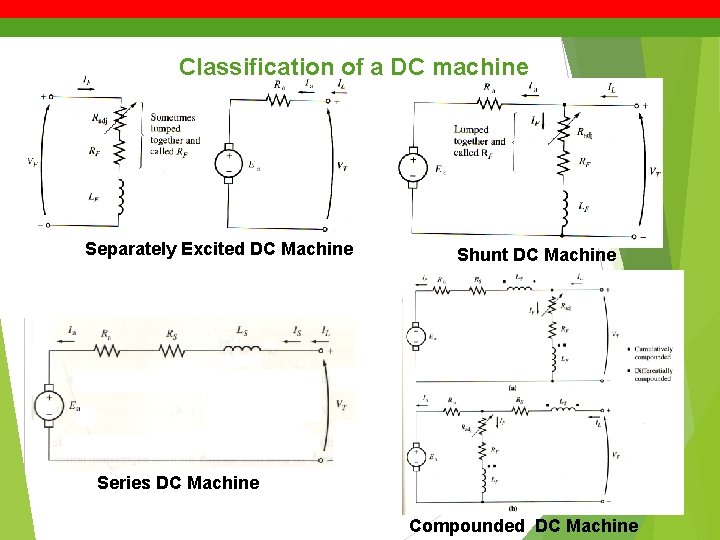
Classification of a DC machine Separately Excited DC Machine Shunt DC Machine Series DC Machine Compounded DC Machine
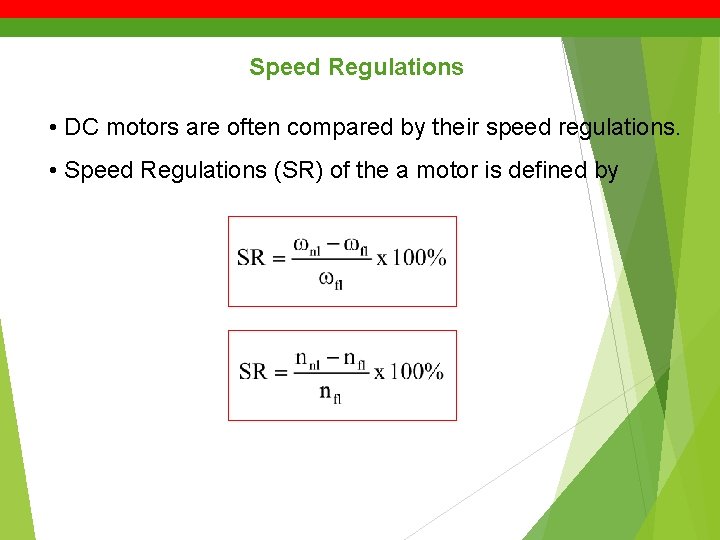
Speed Regulations • DC motors are often compared by their speed regulations. • Speed Regulations (SR) of the a motor is defined by
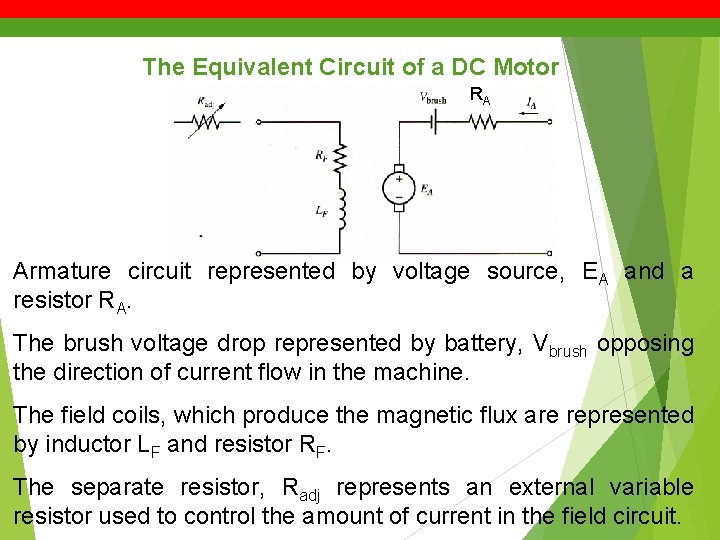
The Equivalent Circuit of a DC Motor RA Armature circuit represented by voltage source, EA and a resistor RA. The brush voltage drop represented by battery, Vbrush opposing the direction of current flow in the machine. The field coils, which produce the magnetic flux are represented by inductor LF and resistor RF. The separate resistor, Radj represents an external variable resistor used to control the amount of current in the field circuit.
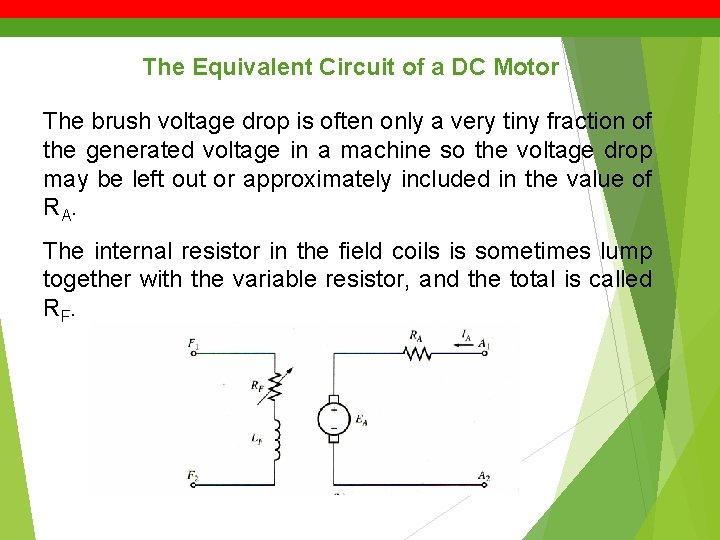
The Equivalent Circuit of a DC Motor The brush voltage drop is often only a very tiny fraction of the generated voltage in a machine so the voltage drop may be left out or approximately included in the value of RA. The internal resistor in the field coils is sometimes lump together with the variable resistor, and the total is called RF.
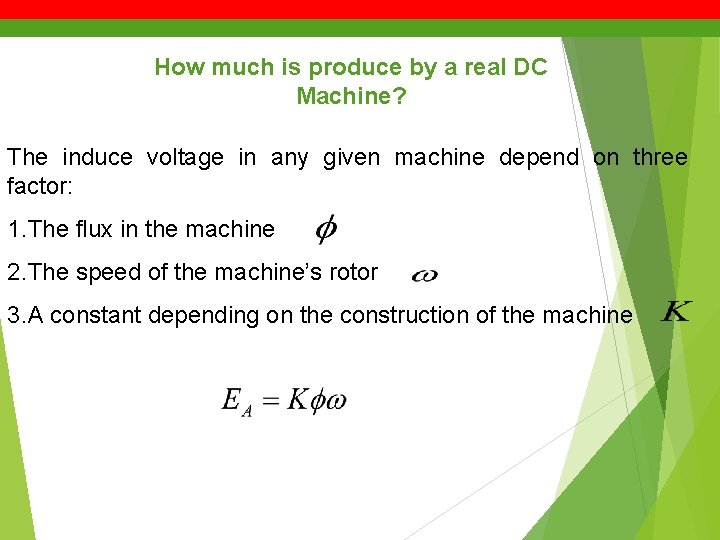
How much is produce by a real DC Machine? The induce voltage in any given machine depend on three factor: 1. The flux in the machine 2. The speed of the machine’s rotor 3. A constant depending on the construction of the machine
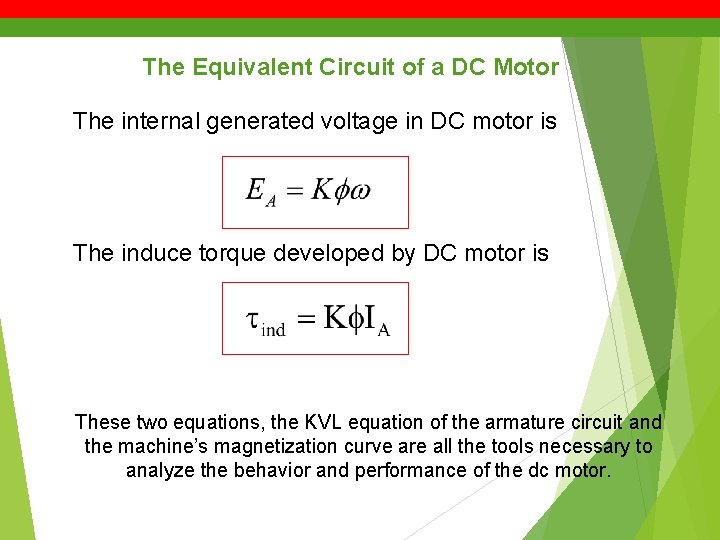
The Equivalent Circuit of a DC Motor The internal generated voltage in DC motor is The induce torque developed by DC motor is These two equations, the KVL equation of the armature circuit and the machine’s magnetization curve are all the tools necessary to analyze the behavior and performance of the dc motor.
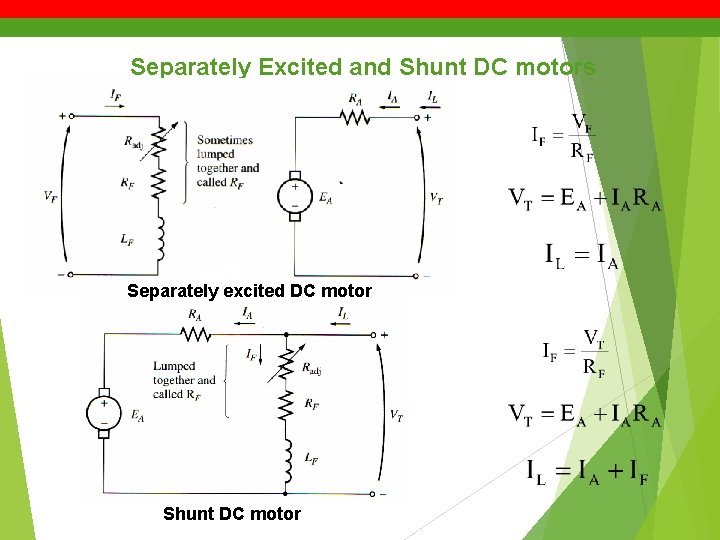
Separately Excited and Shunt DC motors Separately excited DC motor Shunt DC motor
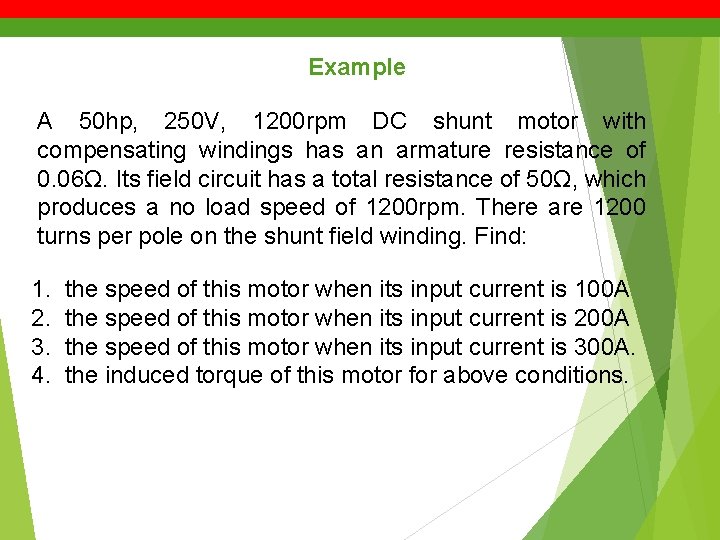
Example A 50 hp, 250 V, 1200 rpm DC shunt motor with compensating windings has an armature resistance of 0. 06Ω. Its field circuit has a total resistance of 50Ω, which produces a no load speed of 1200 rpm. There are 1200 turns per pole on the shunt field winding. Find: 1. 2. 3. 4. the speed of this motor when its input current is 100 A the speed of this motor when its input current is 200 A the speed of this motor when its input current is 300 A. the induced torque of this motor for above conditions.
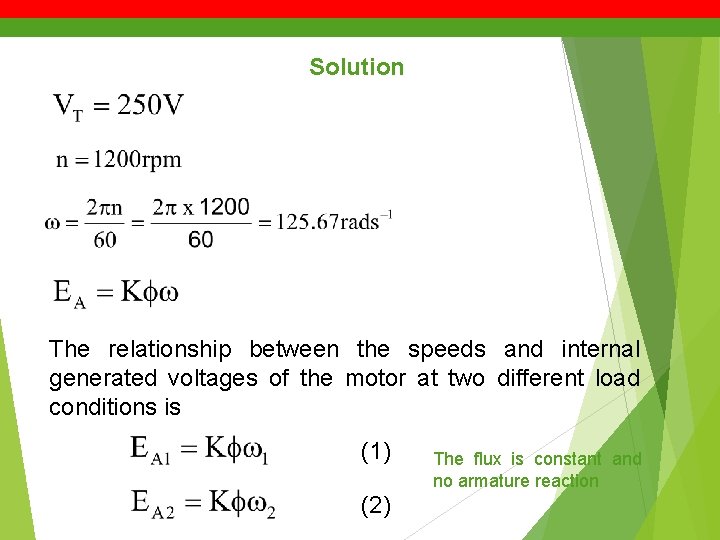
Solution The relationship between the speeds and internal generated voltages of the motor at two different load conditions is (1) (2) The flux is constant and no armature reaction
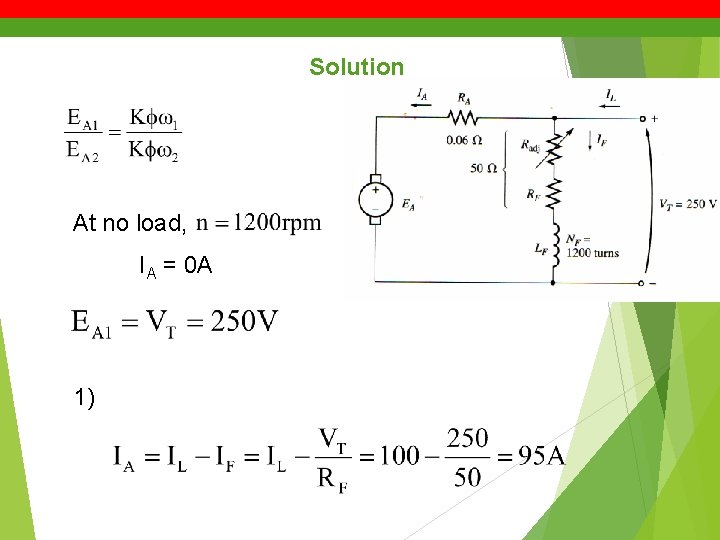
Solution At no load, IA = 0 A 1)
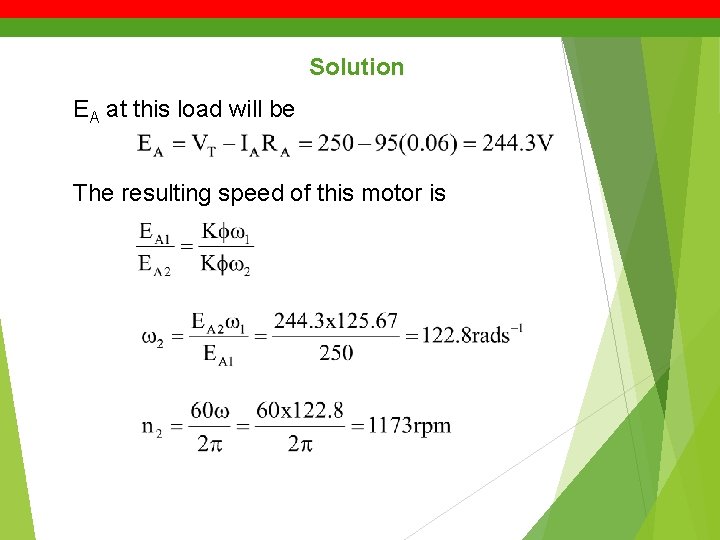
Solution EA at this load will be The resulting speed of this motor is
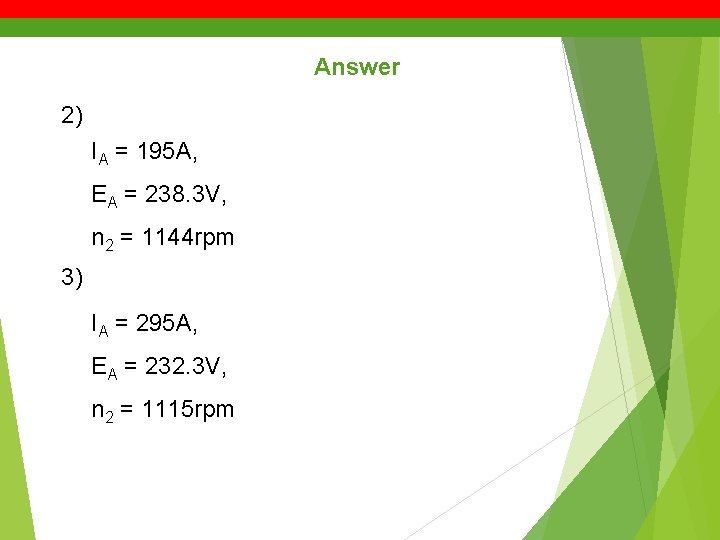
Answer 2) IA = 195 A, EA = 238. 3 V, n 2 = 1144 rpm 3) IA = 295 A, EA = 232. 3 V, n 2 = 1115 rpm
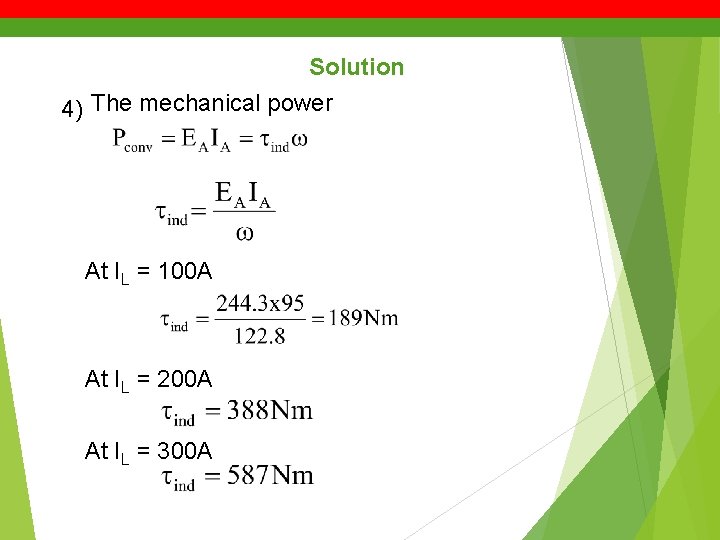
Solution 4) The mechanical power At IL = 100 A At IL = 200 A At IL = 300 A
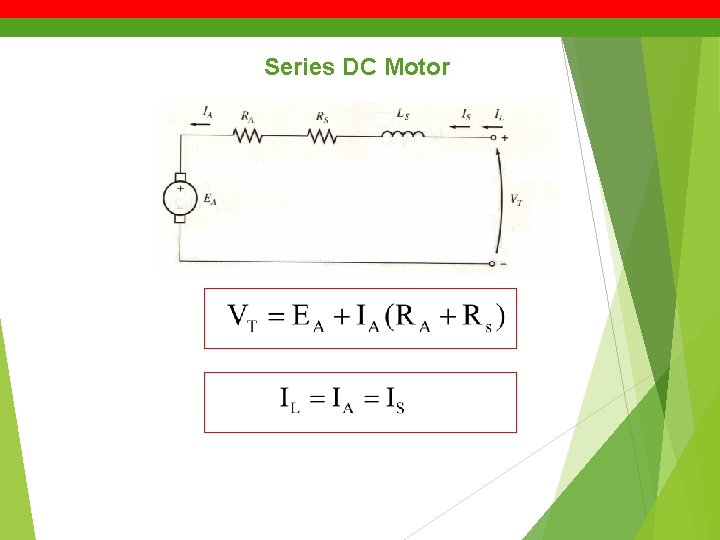
Series DC Motor
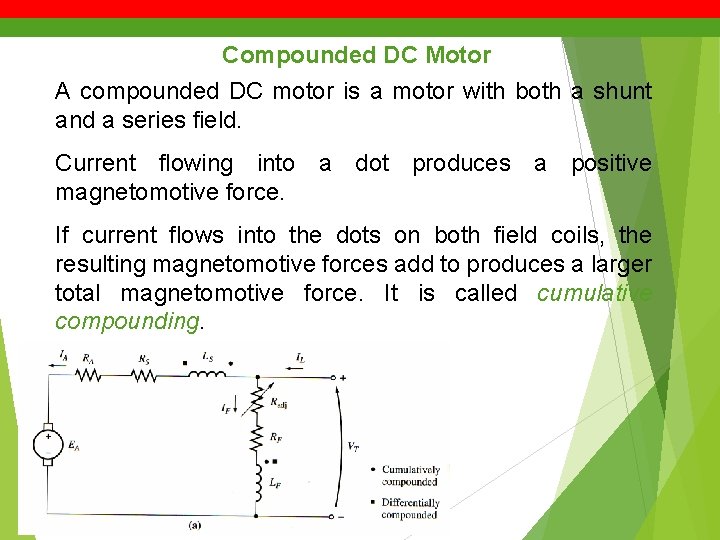
Compounded DC Motor A compounded DC motor is a motor with both a shunt and a series field. Current flowing into a dot produces a positive magnetomotive force. If current flows into the dots on both field coils, the resulting magnetomotive forces add to produces a larger total magnetomotive force. It is called cumulative compounding.
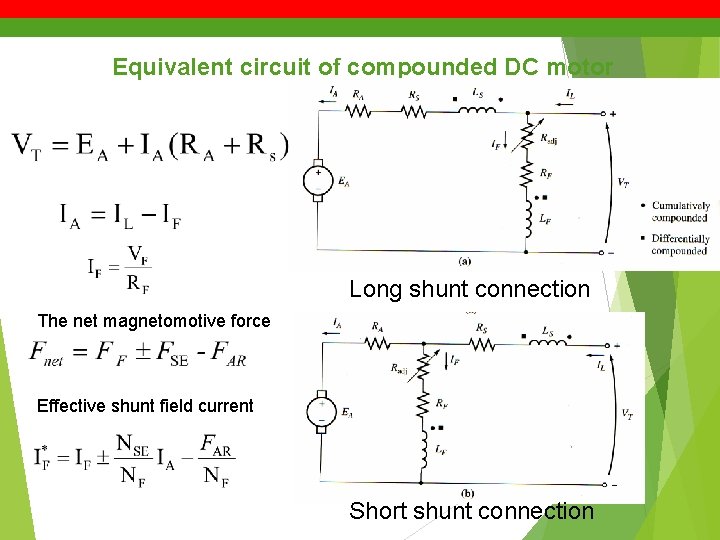
Equivalent circuit of compounded DC motor Long shunt connection The net magnetomotive force Effective shunt field current Short shunt connection
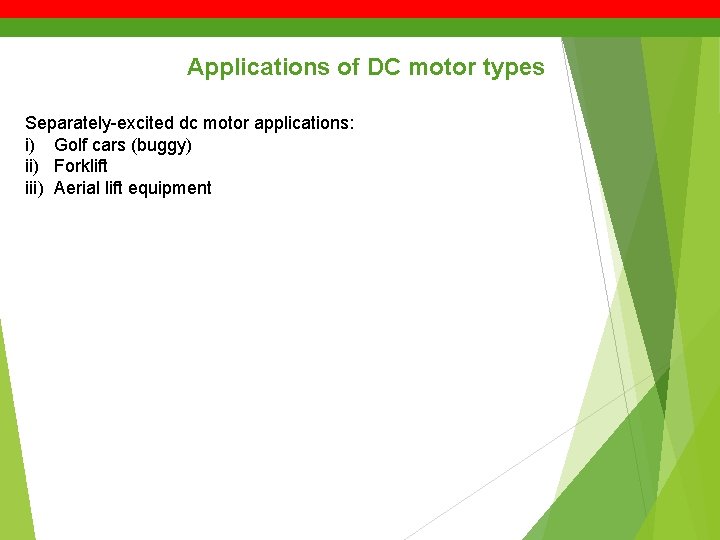
Applications of DC motor types Separately-excited dc motor applications: i) Golf cars (buggy) ii) Forklift iii) Aerial lift equipment
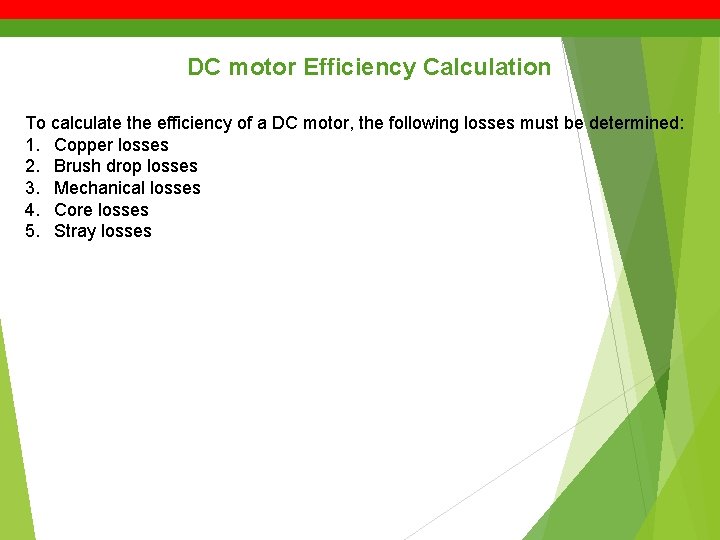
DC motor Efficiency Calculation To calculate the efficiency of a DC motor, the following losses must be determined: 1. Copper losses 2. Brush drop losses 3. Mechanical losses 4. Core losses 5. Stray losses
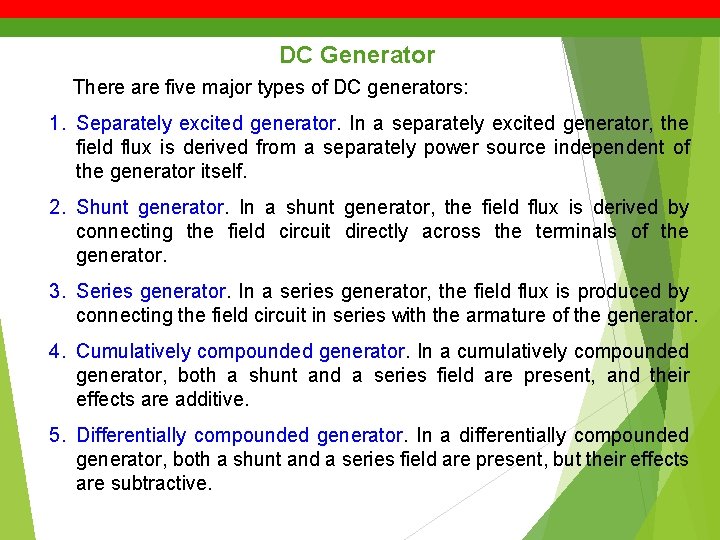
DC Generator There are five major types of DC generators: 1. Separately excited generator. In a separately excited generator, the field flux is derived from a separately power source independent of the generator itself. 2. Shunt generator. In a shunt generator, the field flux is derived by connecting the field circuit directly across the terminals of the generator. 3. Series generator. In a series generator, the field flux is produced by connecting the field circuit in series with the armature of the generator. 4. Cumulatively compounded generator. In a cumulatively compounded generator, both a shunt and a series field are present, and their effects are additive. 5. Differentially compounded generator. In a differentially compounded generator, both a shunt and a series field are present, but their effects are subtractive.
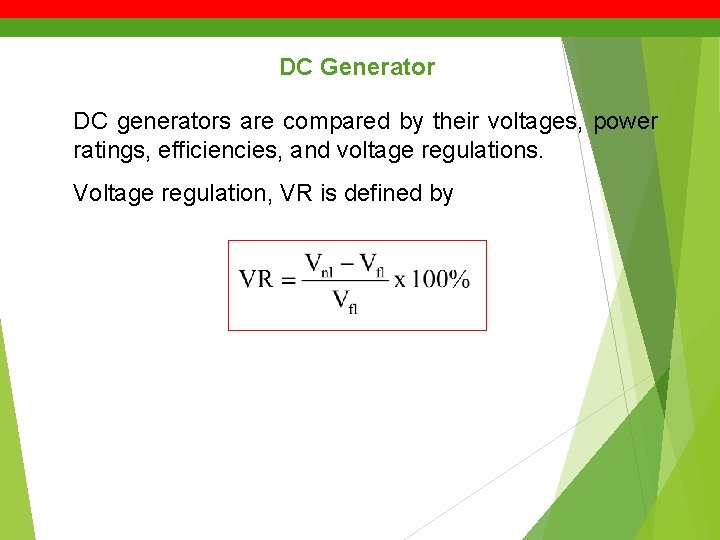
DC Generator DC generators are compared by their voltages, power ratings, efficiencies, and voltage regulations. Voltage regulation, VR is defined by
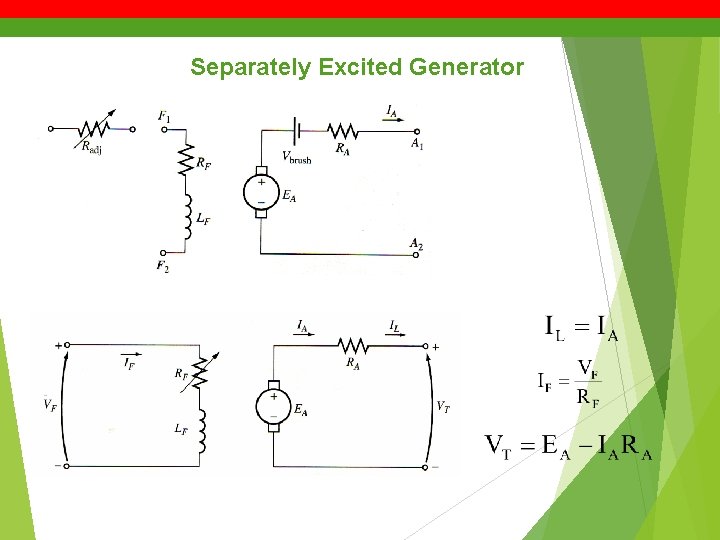
Separately Excited Generator
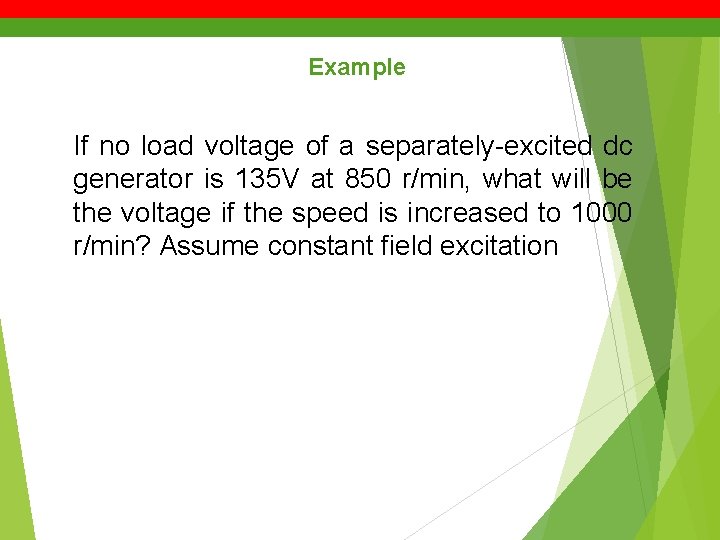
Example If no load voltage of a separately-excited dc generator is 135 V at 850 r/min, what will be the voltage if the speed is increased to 1000 r/min? Assume constant field excitation
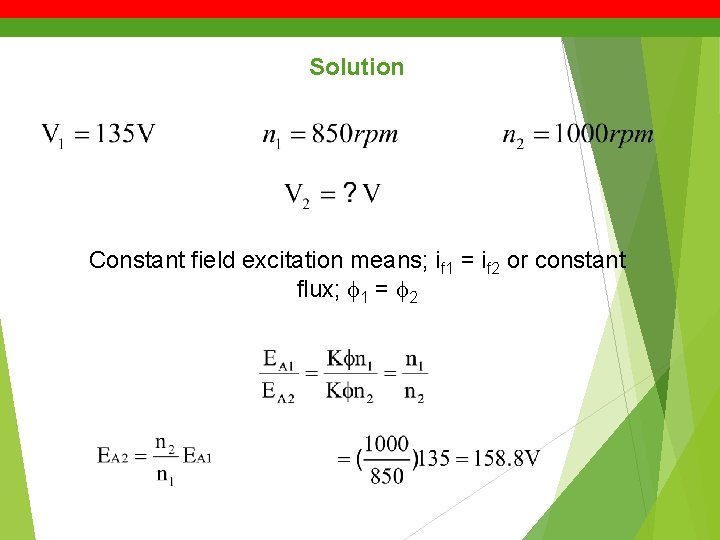
Solution Constant field excitation means; if 1 = if 2 or constant flux; 1 = 2
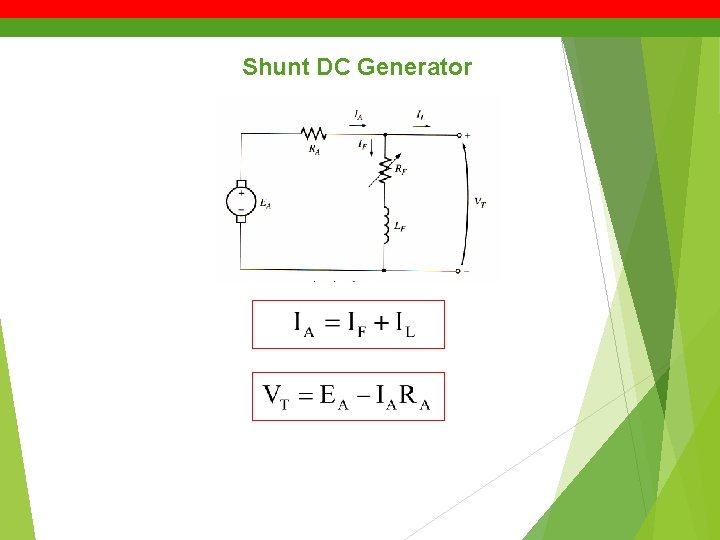
Shunt DC Generator
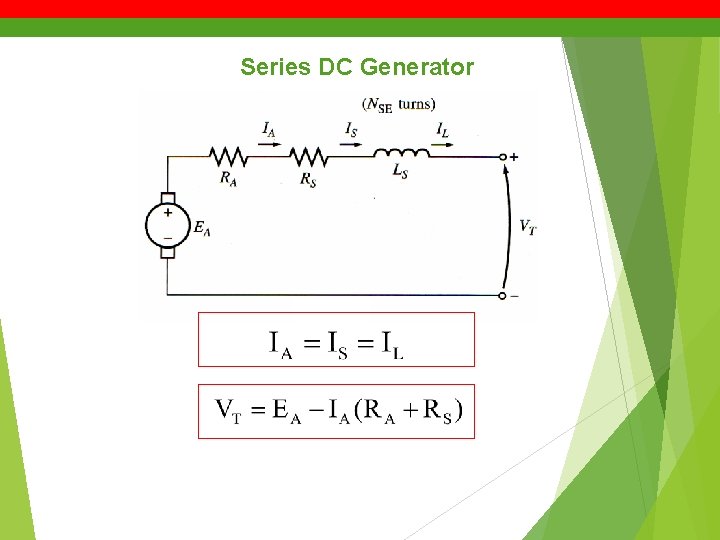
Series DC Generator
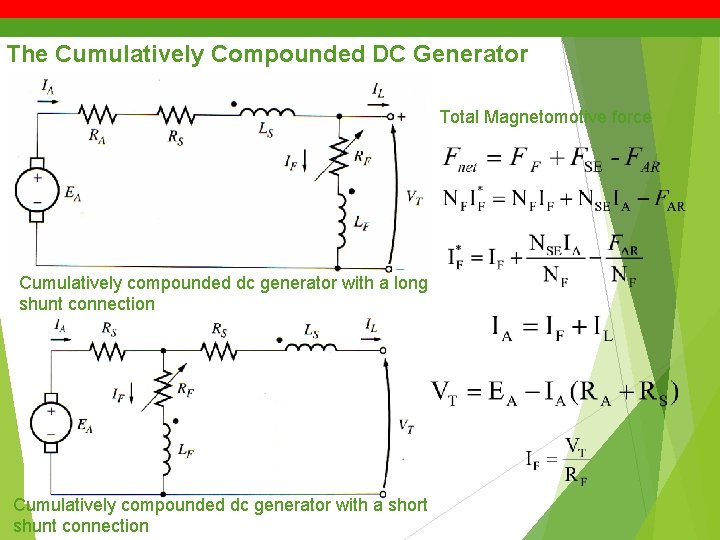
The Cumulatively Compounded DC Generator Total Magnetomotive force Cumulatively compounded dc generator with a long shunt connection Cumulatively compounded dc generator with a short shunt connection
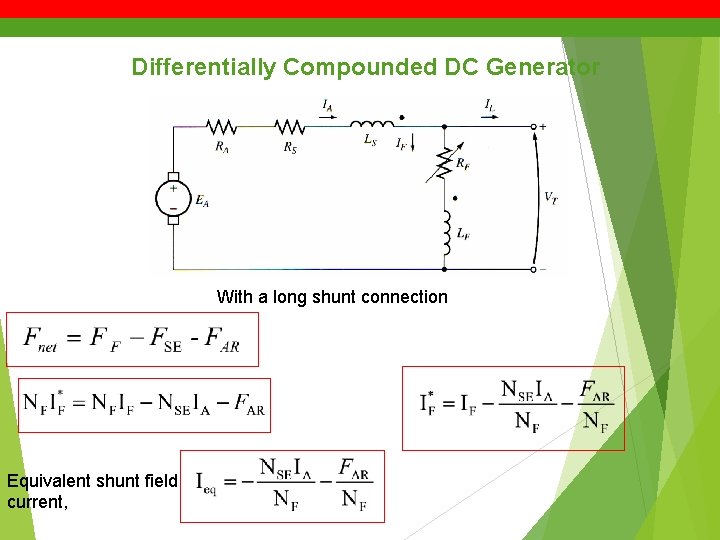
Differentially Compounded DC Generator With a long shunt connection Equivalent shunt field current,
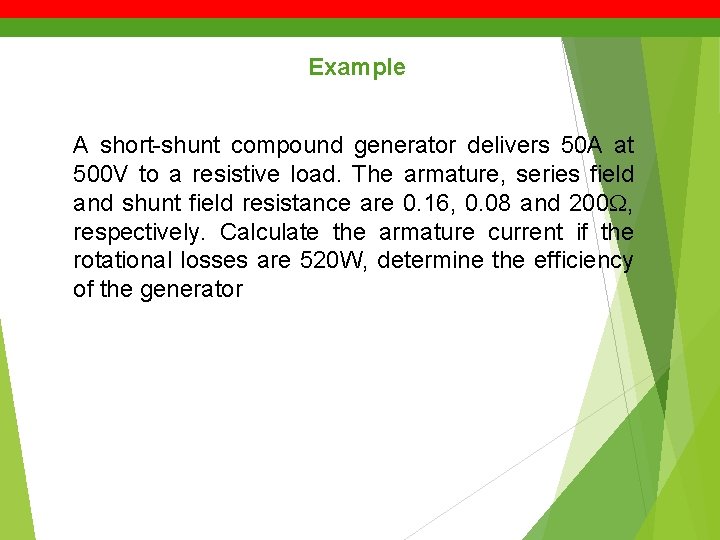
Example A short-shunt compound generator delivers 50 A at 500 V to a resistive load. The armature, series field and shunt field resistance are 0. 16, 0. 08 and 200 , respectively. Calculate the armature current if the rotational losses are 520 W, determine the efficiency of the generator
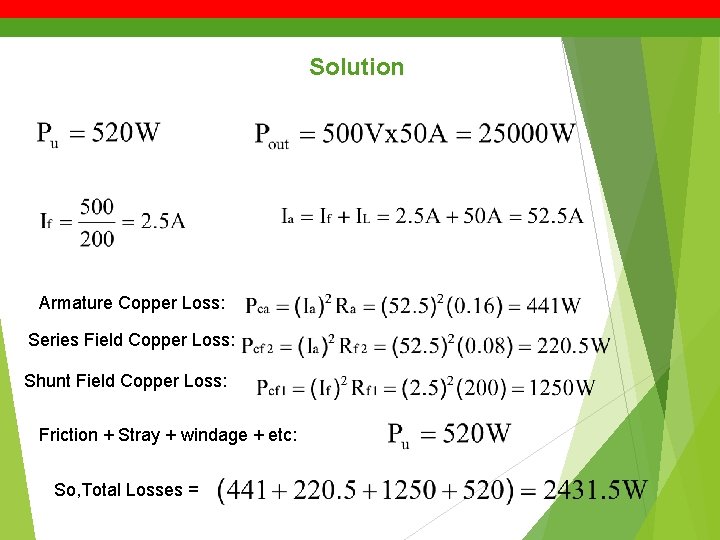
Solution Armature Copper Loss: Series Field Copper Loss: Shunt Field Copper Loss: Friction + Stray + windage + etc: So, Total Losses =
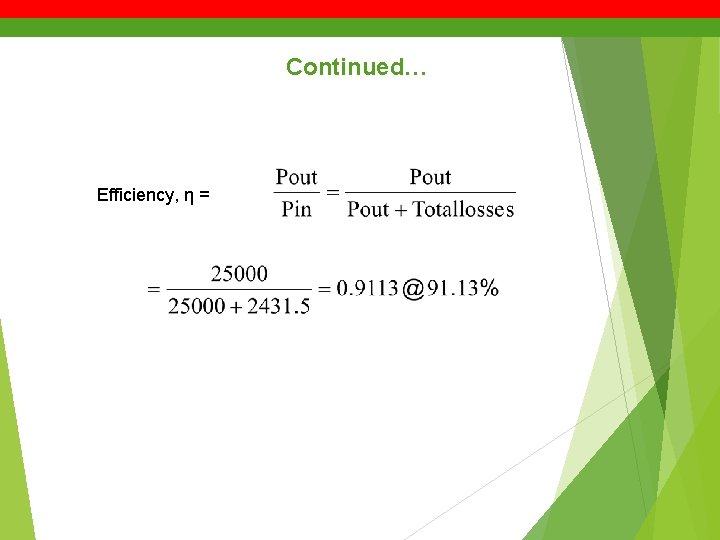
Continued… Efficiency, η =
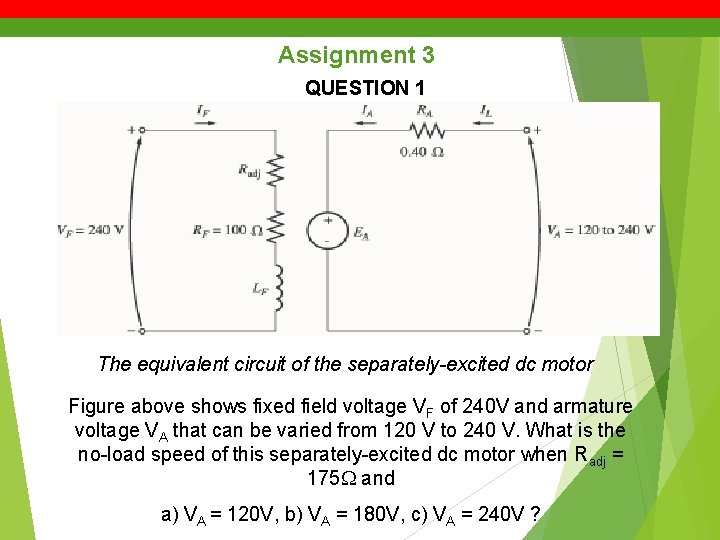
Assignment 3 QUESTION 1 The equivalent circuit of the separately-excited dc motor Figure above shows fixed field voltage VF of 240 V and armature voltage VA that can be varied from 120 V to 240 V. What is the no-load speed of this separately-excited dc motor when Radj = 175 and a) VA = 120 V, b) VA = 180 V, c) VA = 240 V ?
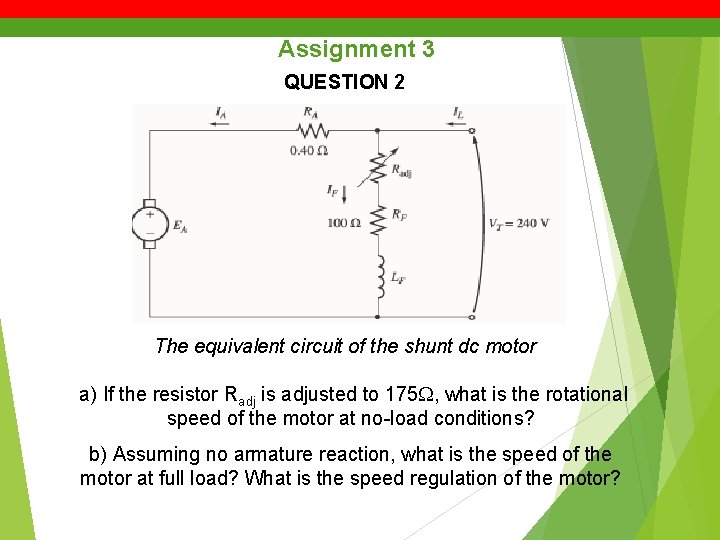
Assignment 3 QUESTION 2 The equivalent circuit of the shunt dc motor a) If the resistor Radj is adjusted to 175 , what is the rotational speed of the motor at no-load conditions? b) Assuming no armature reaction, what is the speed of the motor at full load? What is the speed regulation of the motor?
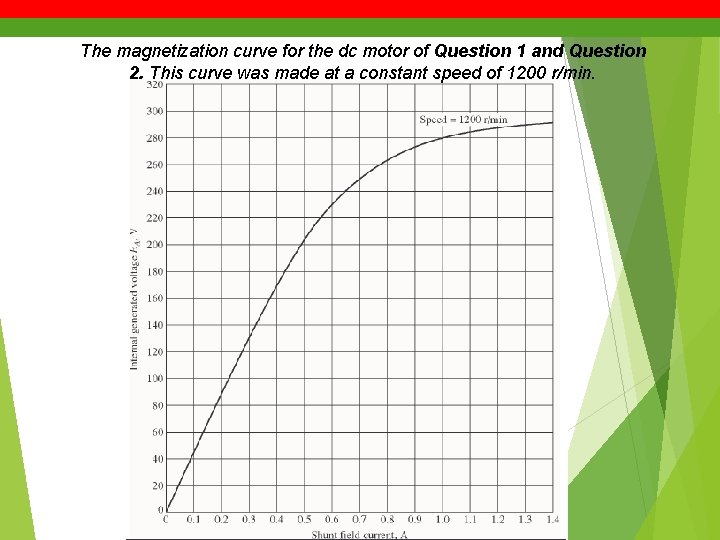
The magnetization curve for the dc motor of Question 1 and Question 2. This curve was made at a constant speed of 1200 r/min.
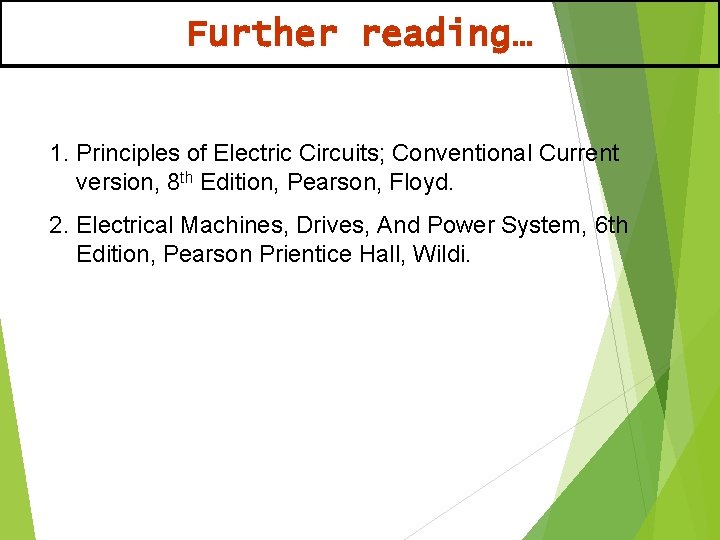
Further reading… 1. Principles of Electric Circuits; Conventional Current version, 8 th Edition, Pearson, Floyd. 2. Electrical Machines, Drives, And Power System, 6 th Edition, Pearson Prientice Hall, Wildi.
Dm 249
Artigo 249 cc
Daftar cek tak laku
Comp 249
P-249 form
Ee 249
Ee 249
Circular susep 249
Lesson 5: electrical nonmetallic tubing (ent)
Incomplete circuit
Venn diagram series and parallel circuits
The circulatory system
Brass paper fastener conductor or insulator
V
Types of circuit
Phet circuit construction kit
Series vs parallel
Parallel short circuit
Series parallel circuit current
Electrical circuit politeknik
Branch node
Live neutral earth symbols
Circuit analysis laplace transform
Electrical circuit analysis
Electric circuit consists of
What is an electrical load
Flashlight electrical circuit
Electrical circuit
S domain circuit analysis
Electrical circuit elements
Basic electrical circuit
Rope model electric circuit
Simple electric circuit and label the parts
An electrical circuit contains at minimum a
Moore machine and mealy machine
Energy work and simple machines chapter 10 answers
Stirred tank bioreactor advantages and disadvantages
Microscope rotating nosepiece
Large scale rotating air mass
Bodytube microscope
The parts of compound microscope
Suzie sees bob rotating clockwise
Rotating conic sections
Balancing of several masses rotating in the same plane
Rotary system components
Body parts of microscope
Instantaneous value of the emf phasor
Rotating shaft design
Zqna
Relative motion analysis using rotating axes
Compared to a gear tooth on the rear sprocket
Compound microscope function
Mechanical parts of microscope
Non rotating planet
Pillar microscope function
Culture definition
Locomotor definition in dance
A disk initially rotating at 120 rad/s
Rotating cube display
Types of congruence transformation
Todd f. heatherton
Main function of bearing
Where can we find rotating arm sprays in sewage treatment?
Adelsons checkerboard
Front wheel drive tire rotation
Moment of inertia hoop
Right hand rule for rotation
Rotating coordinate systems
Rigid body transformation in computer graphics
Spinning ballerina illusion
Heel effect
Chapter 31 faraday law ofinduction
Rotating lidar
This yes
Circular motion
Rotational motion equations
Clamp and drill hydraulic circuit
Suction machine parts and function pdf
Tens machine circuit diagram
Electric machine design
Finite state machine vending machine example
Mealy to moore conversion
Dr max klein ent
Template
Ent guynemer toulouse
Ent raymond naves
Ent jean monnet aubigny en artois
Des lauselühend
Ent163
Ent 163
Ent.163
Ent 163
Ent 163
Ent uh1
Ent fellowship europe
Ent constant boudoux
Ent 407
Ent 22010
Ent 163
Ent ulco