DNA Protein Synthesis The Major Players Their Work
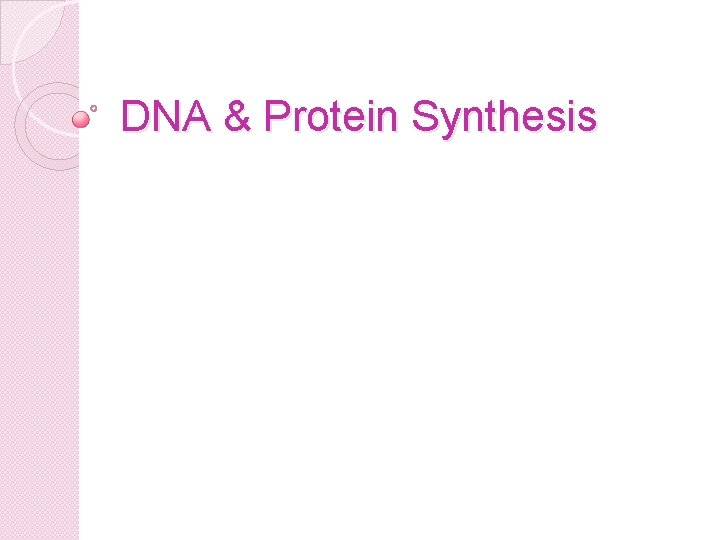
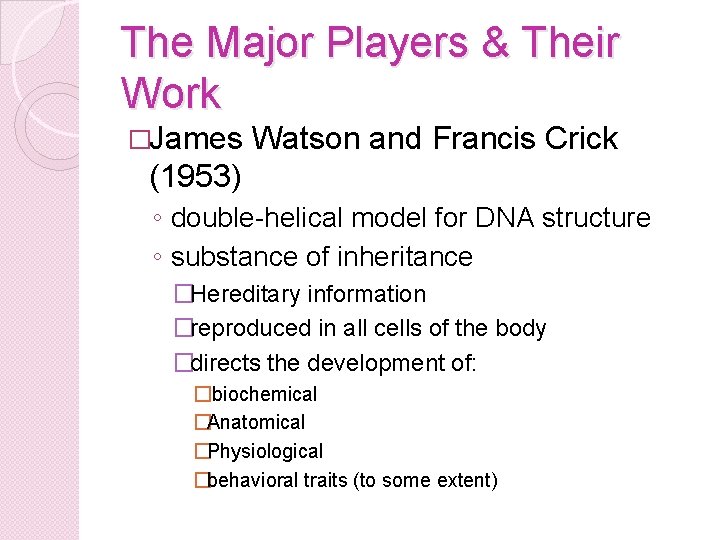
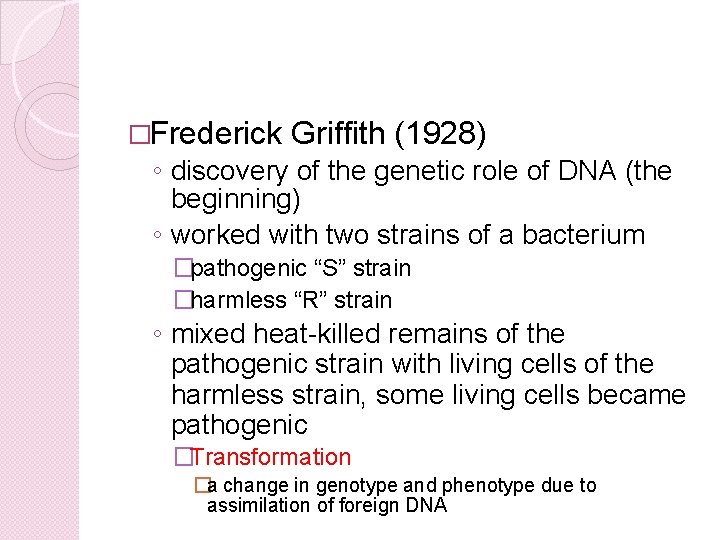
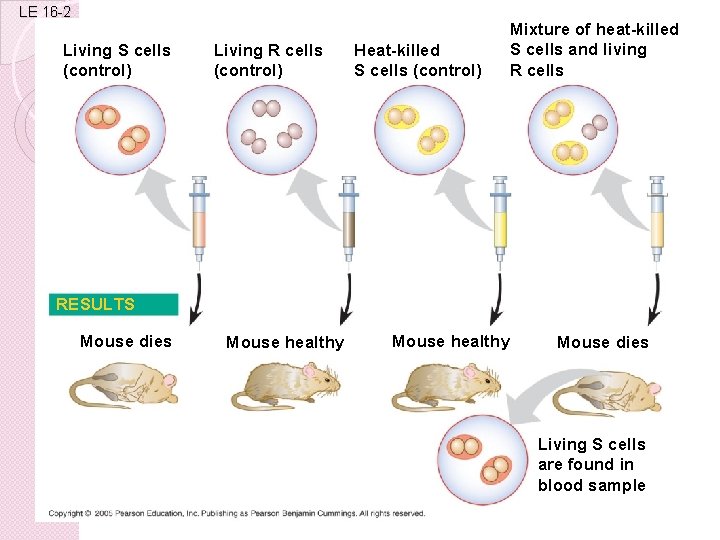
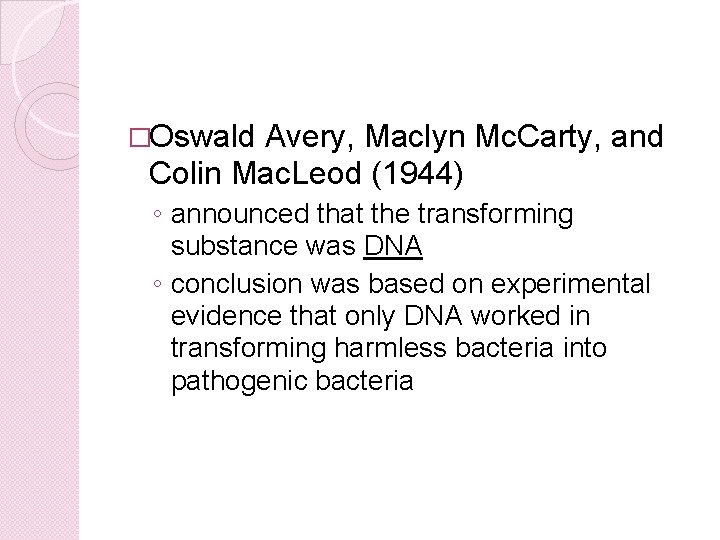
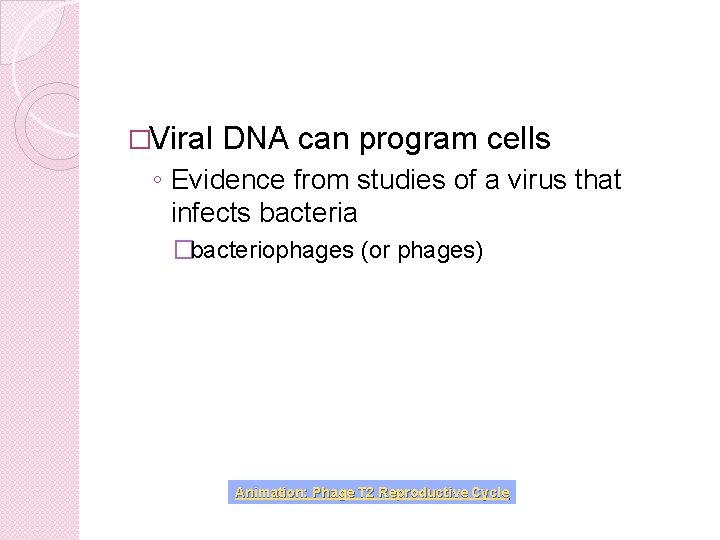
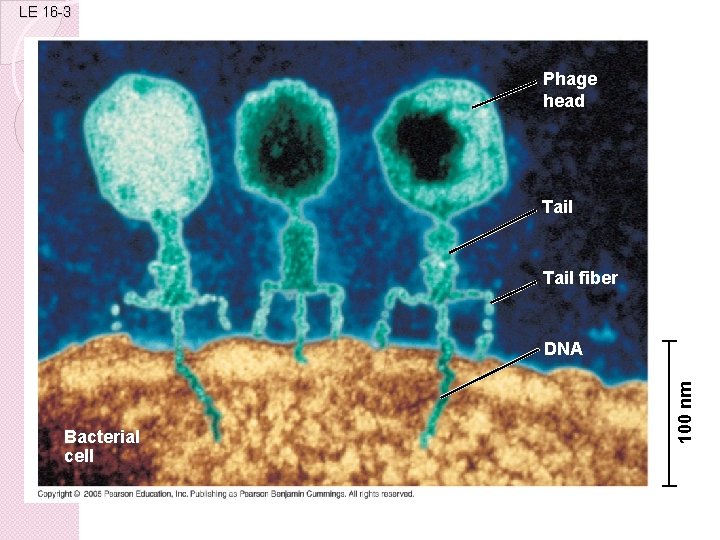
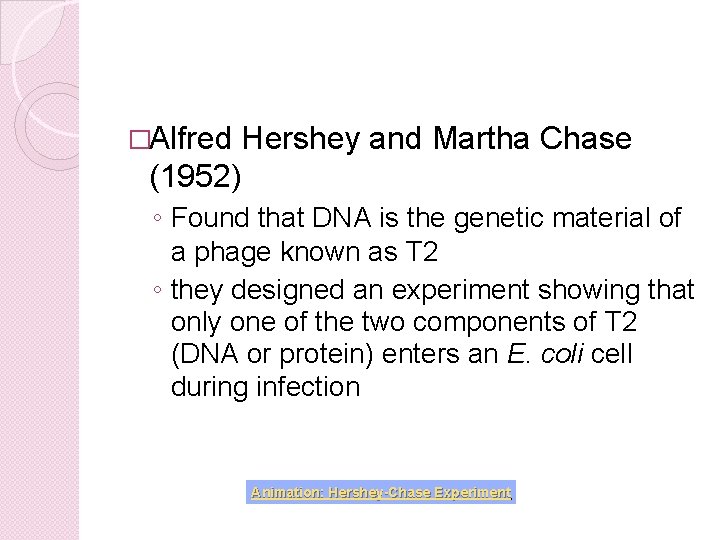
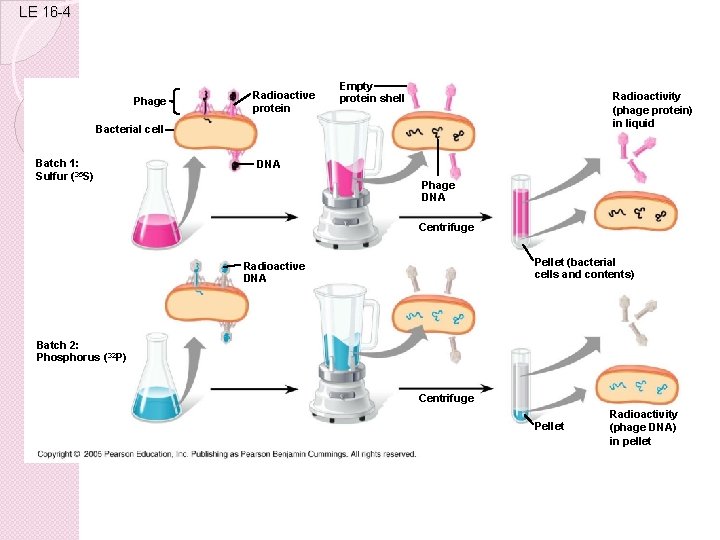
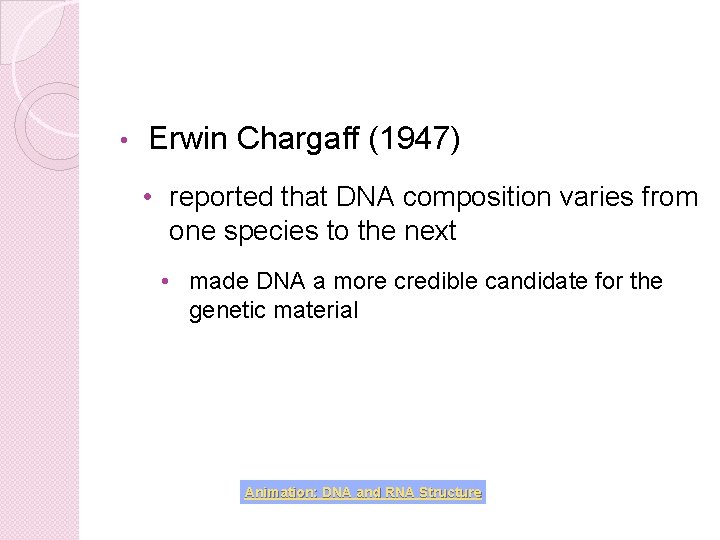
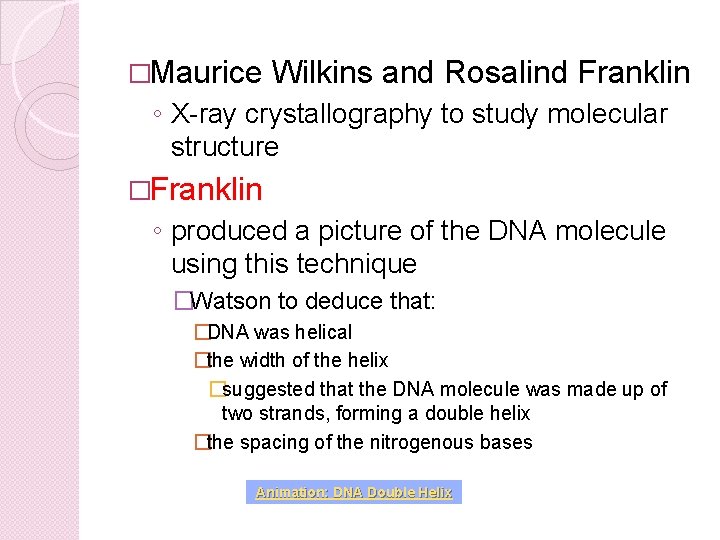
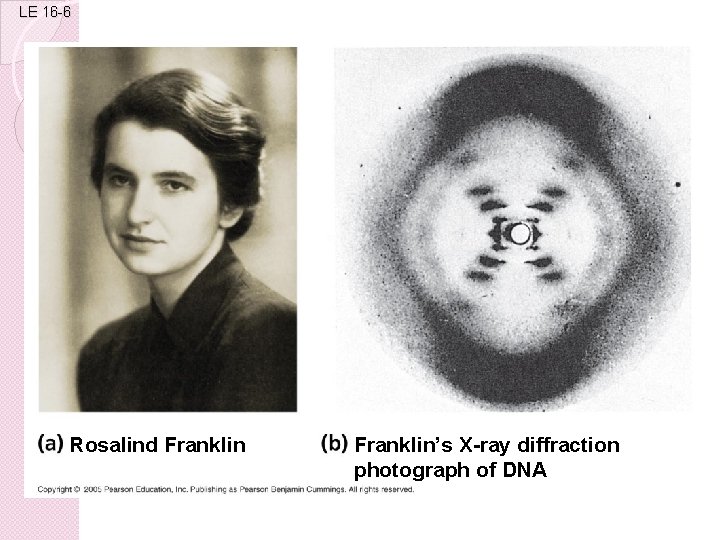
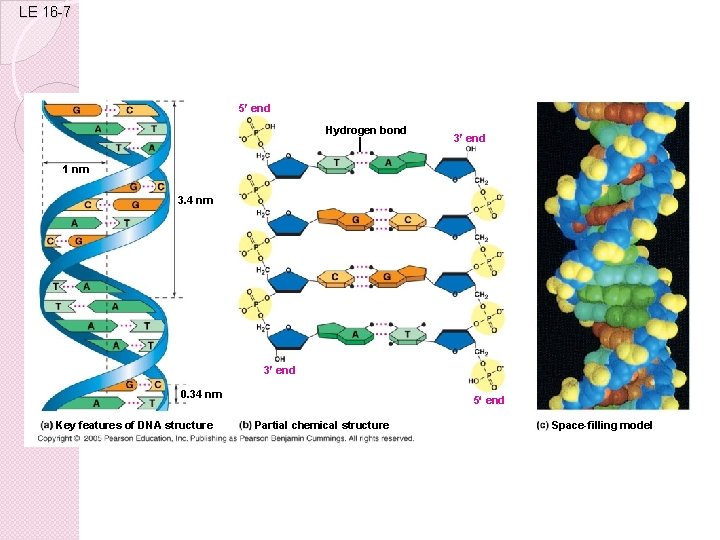
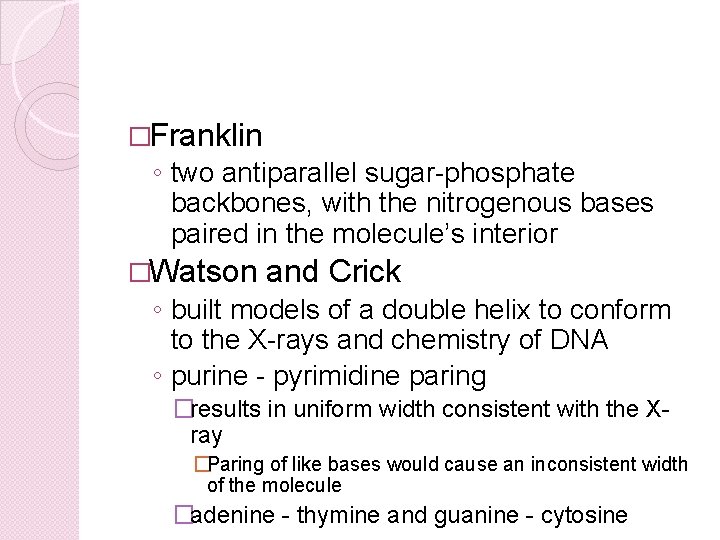
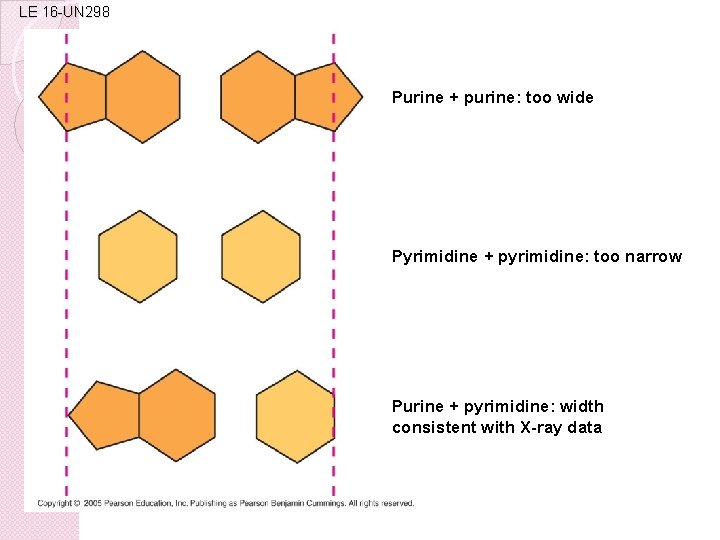
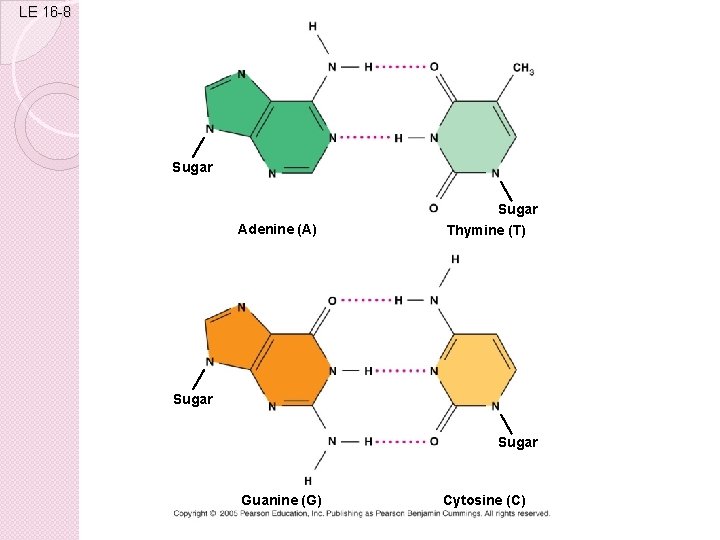
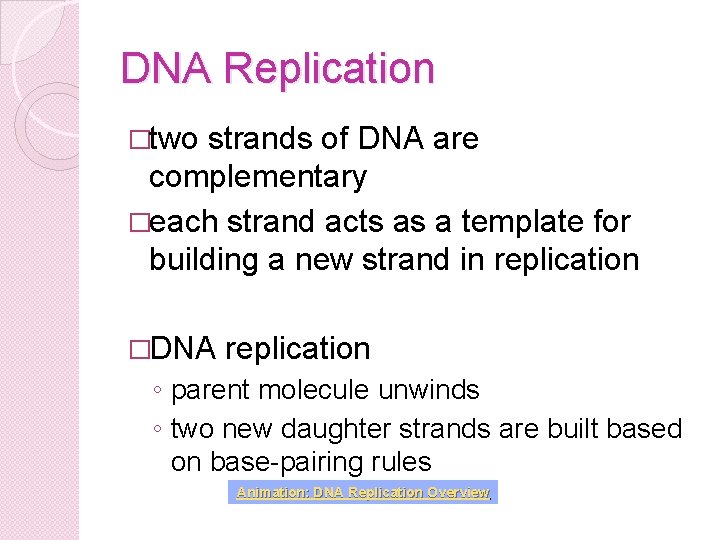
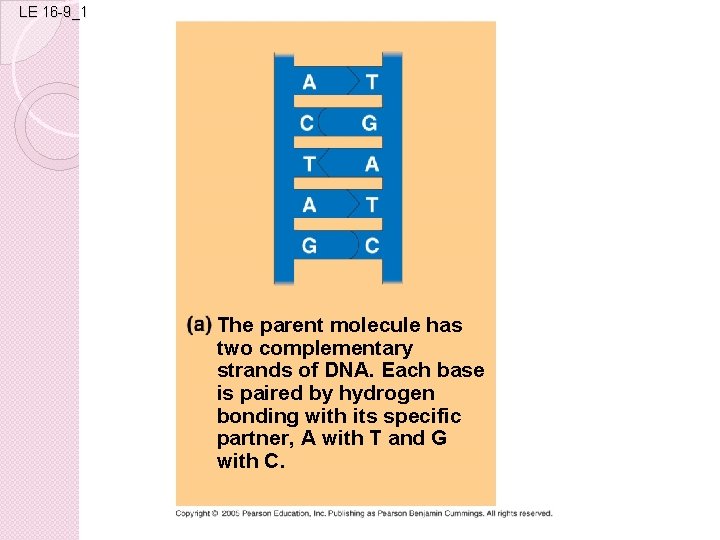
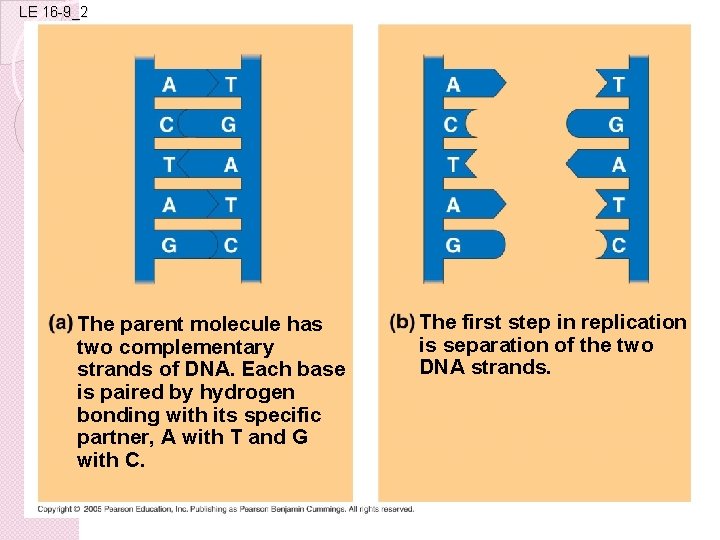
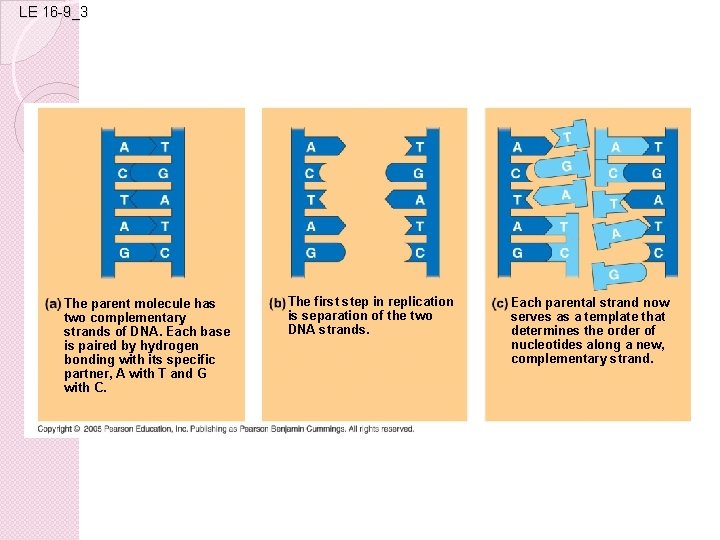
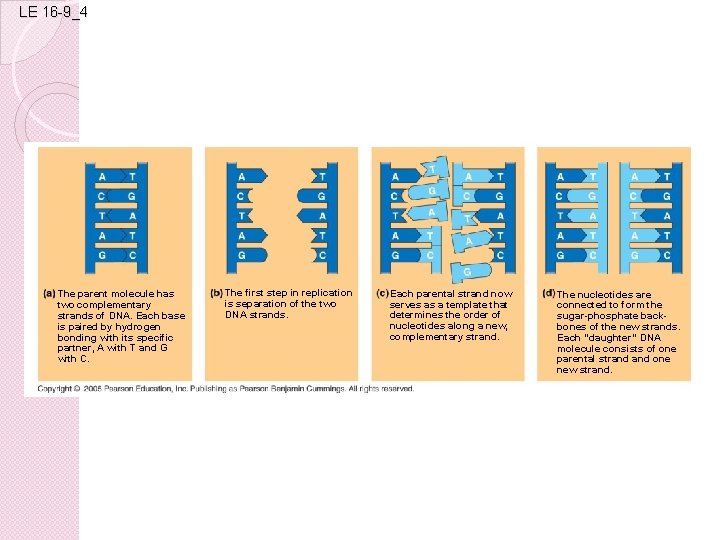
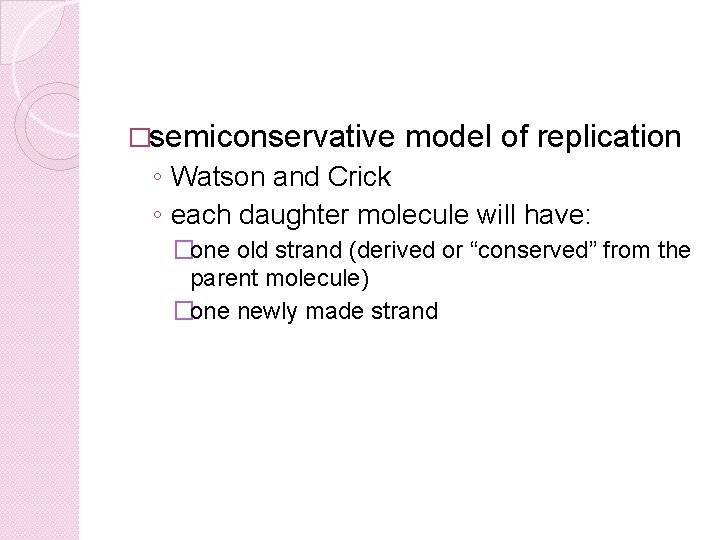
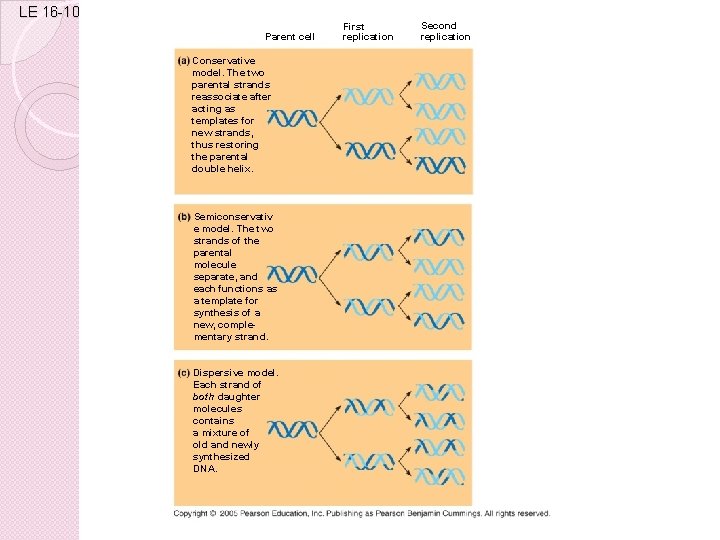
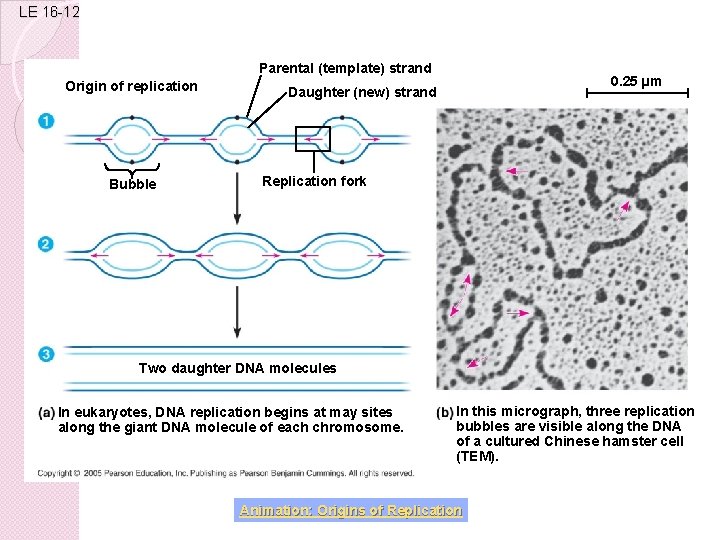
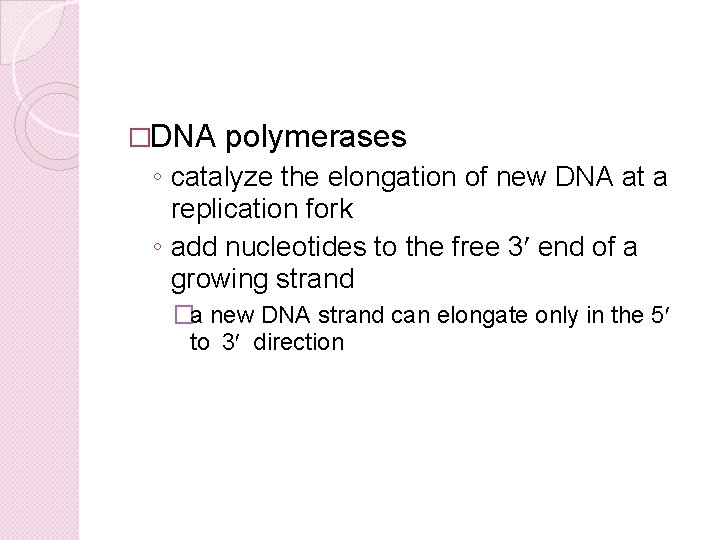
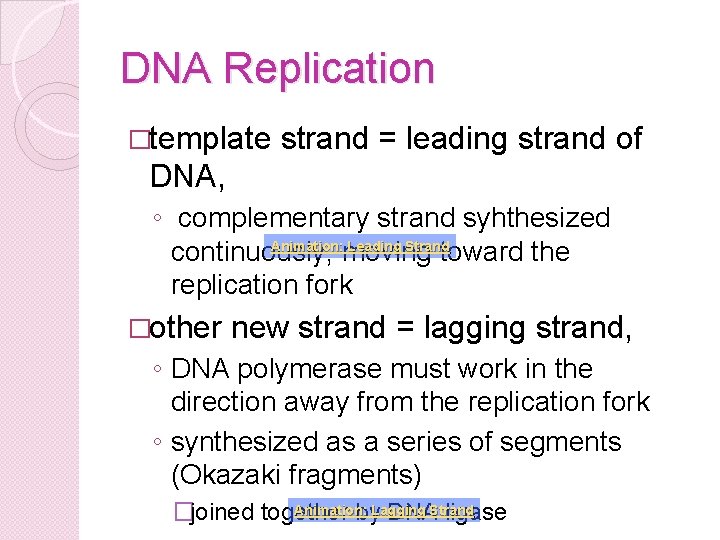
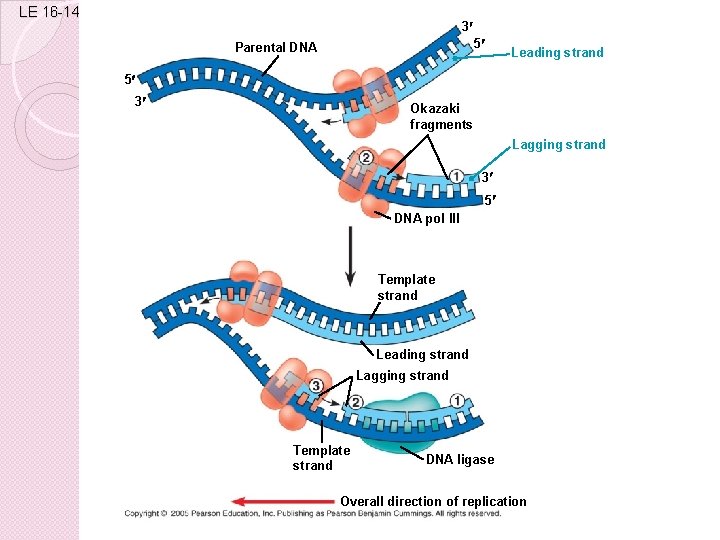
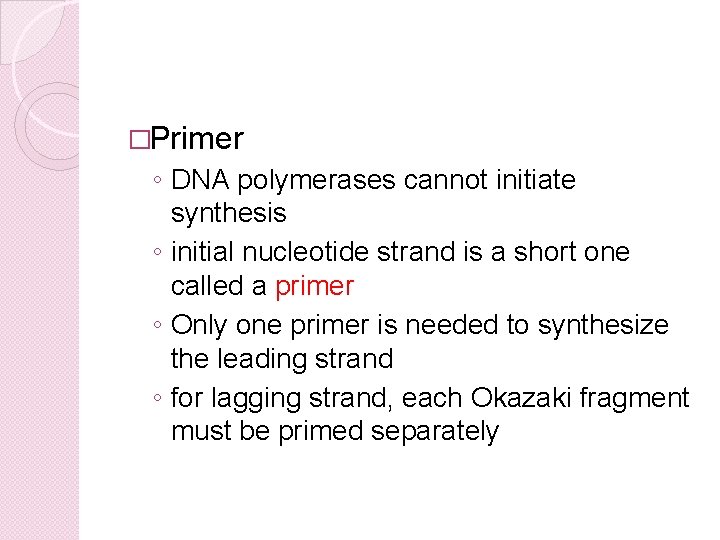
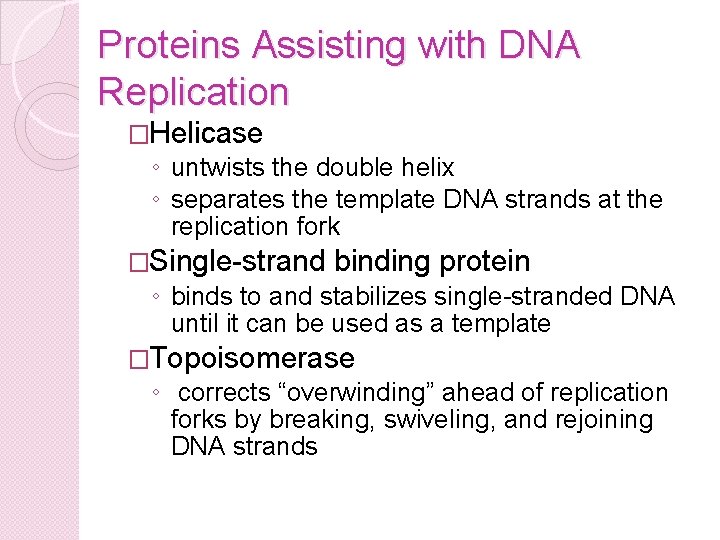
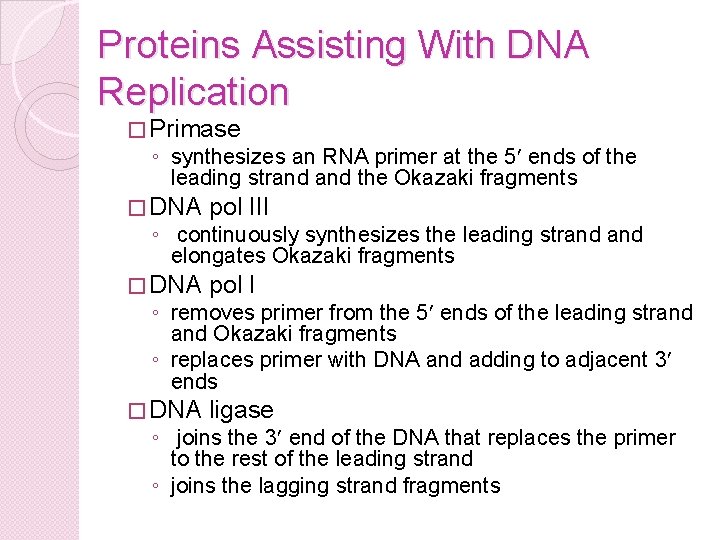
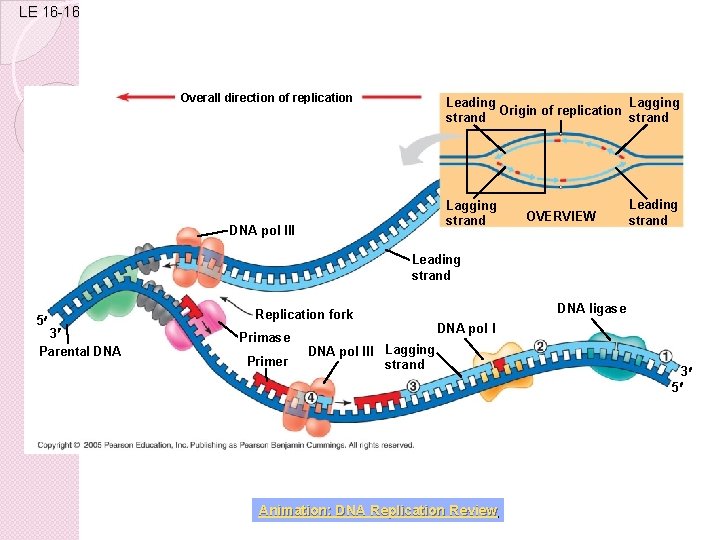
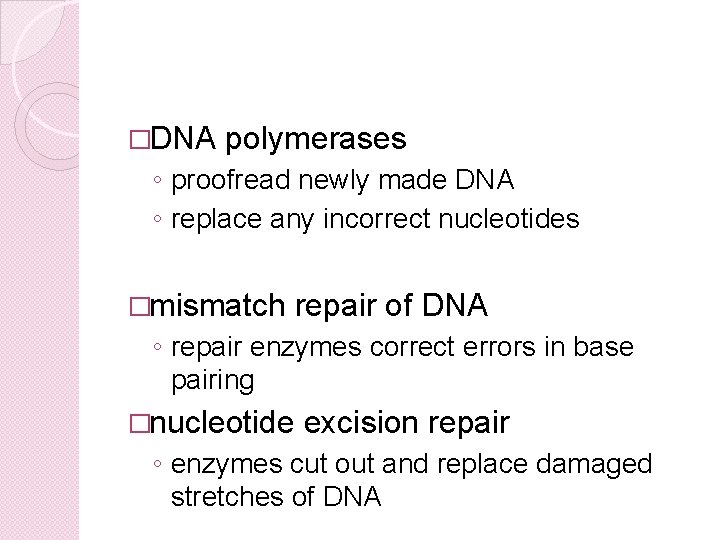
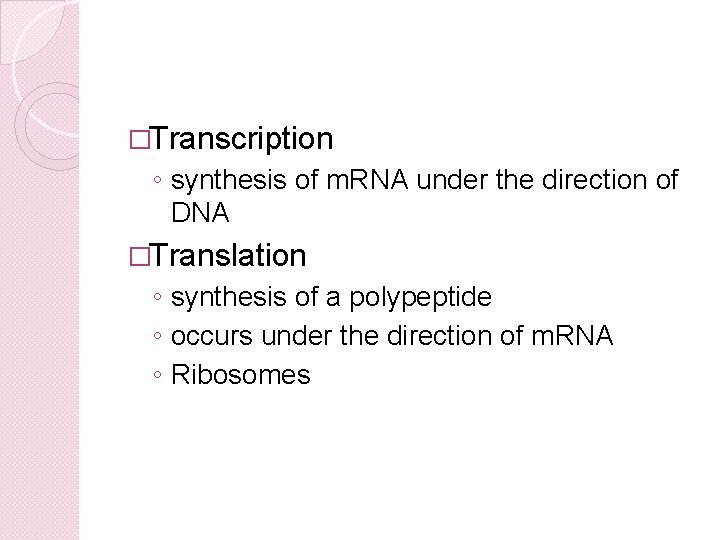
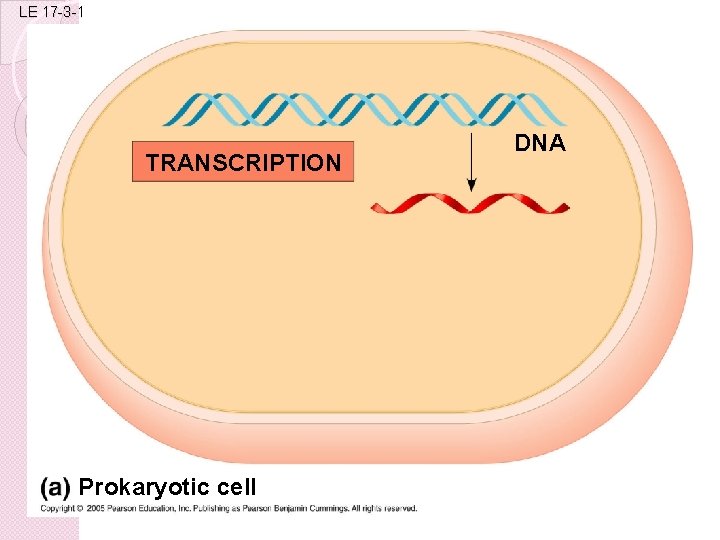
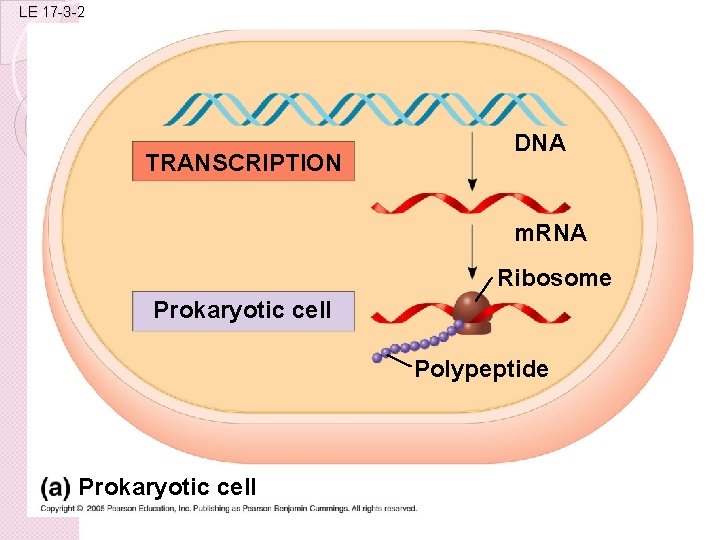
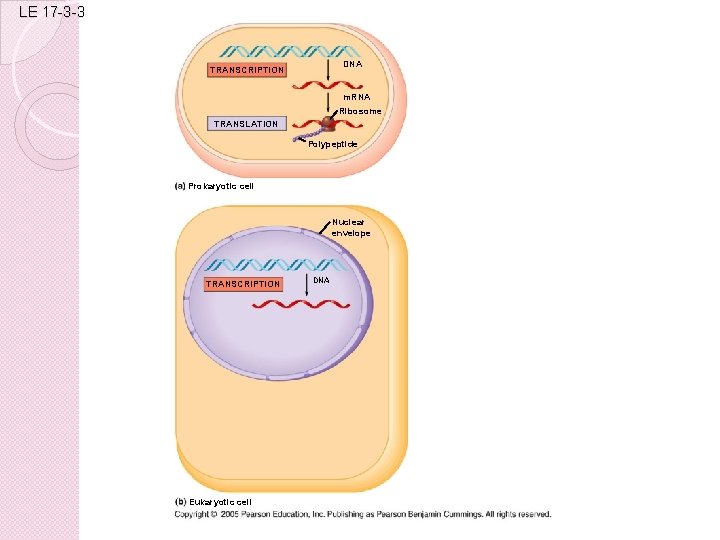
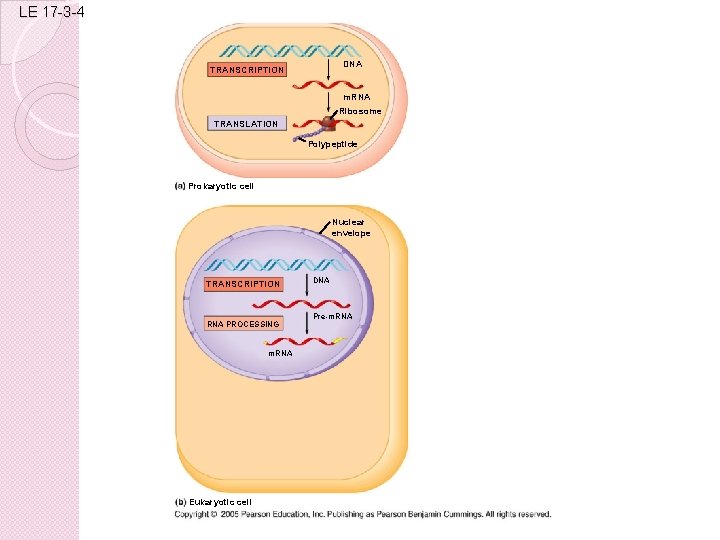
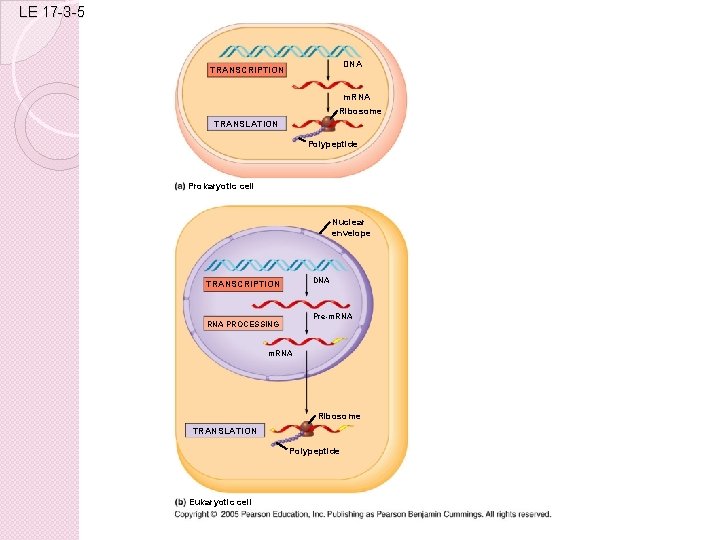
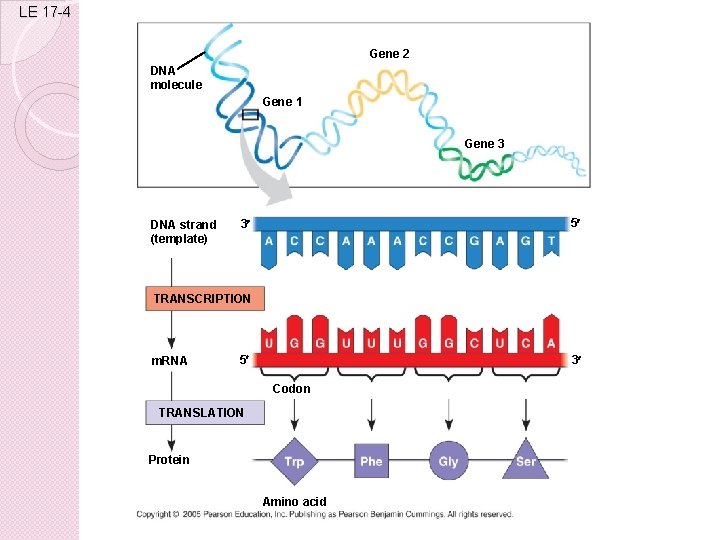
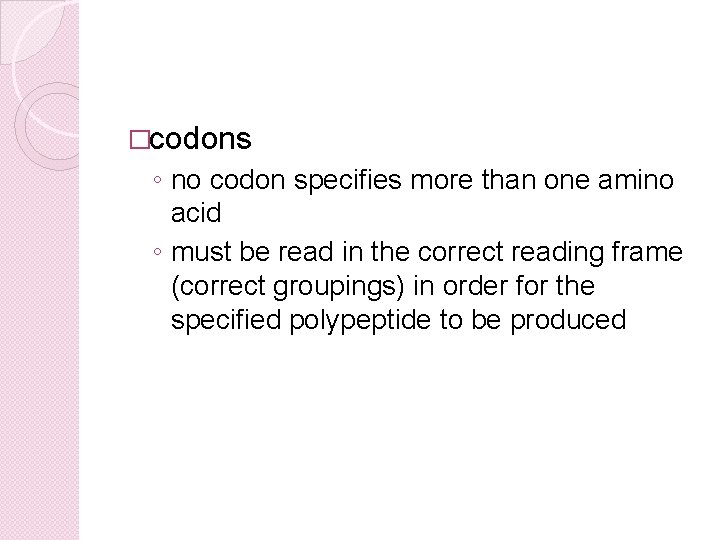
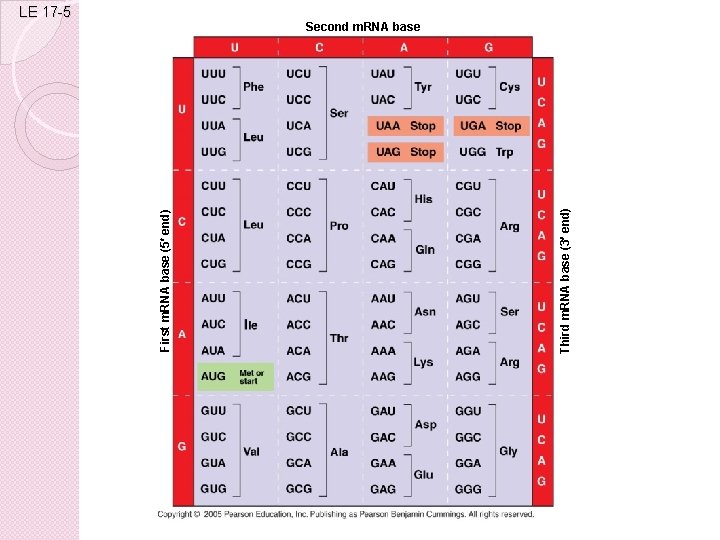
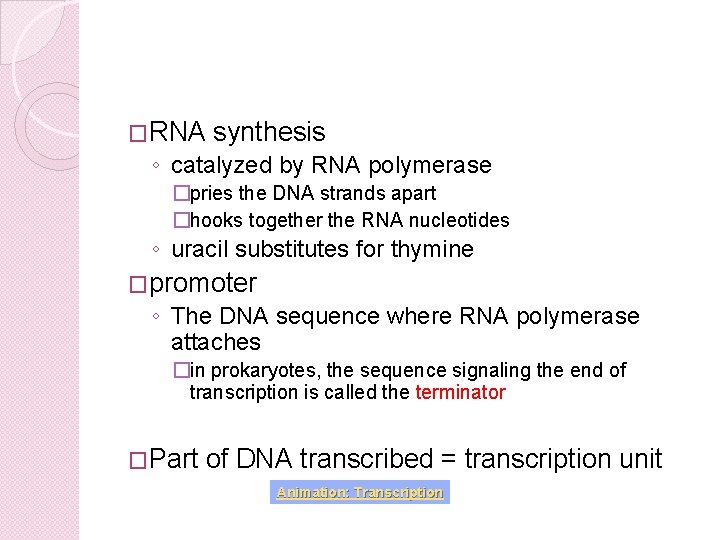
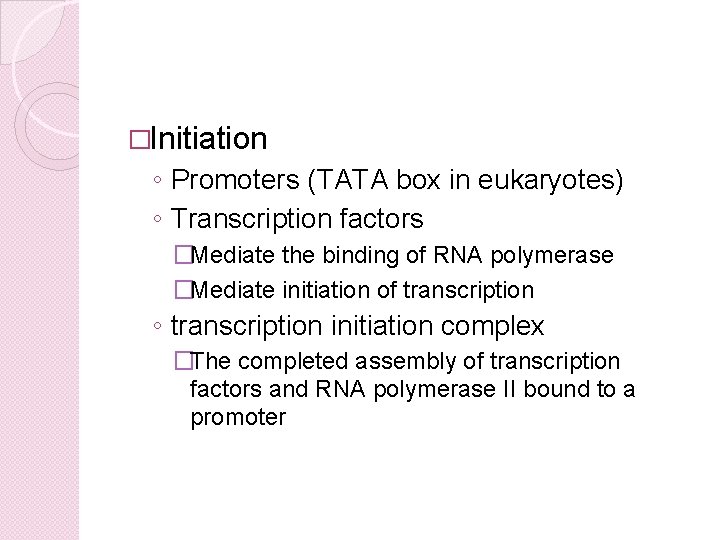
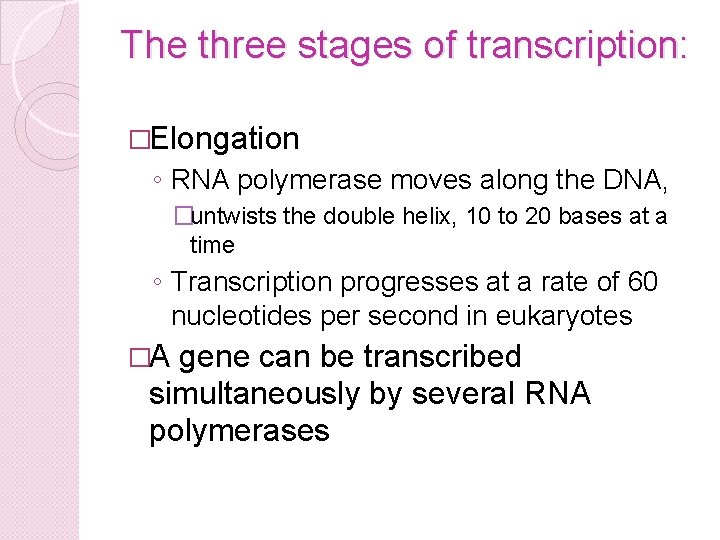
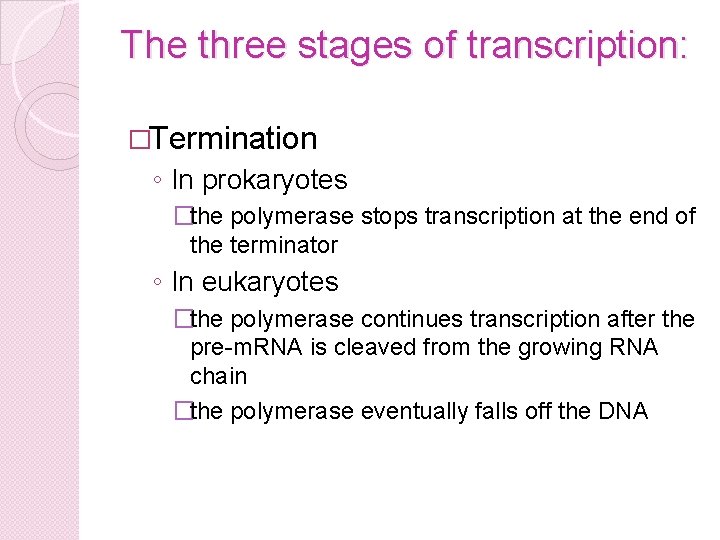
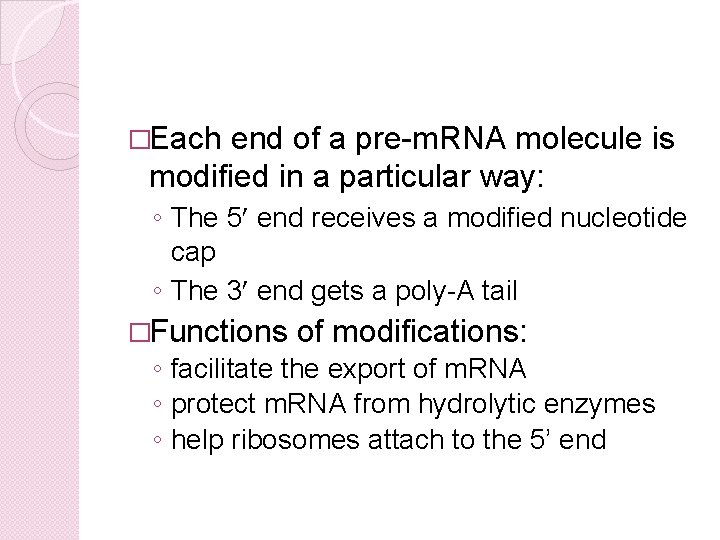
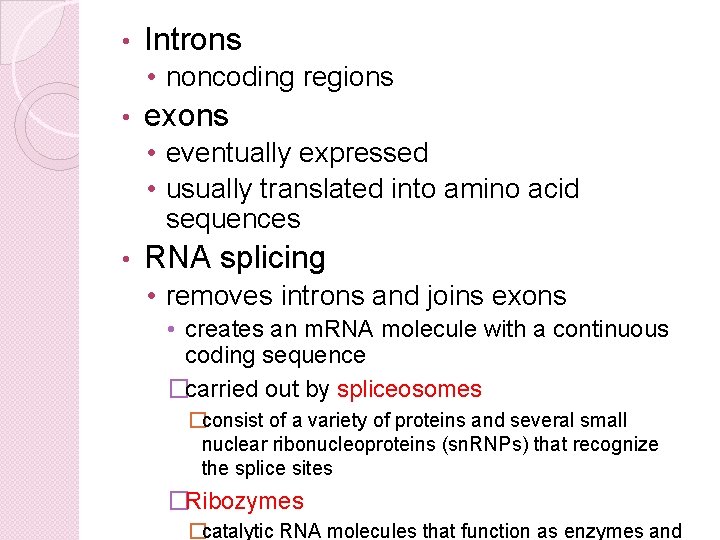
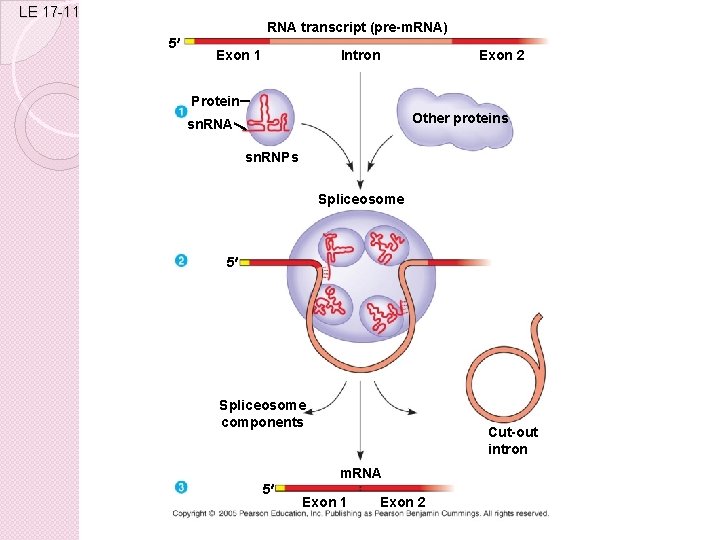
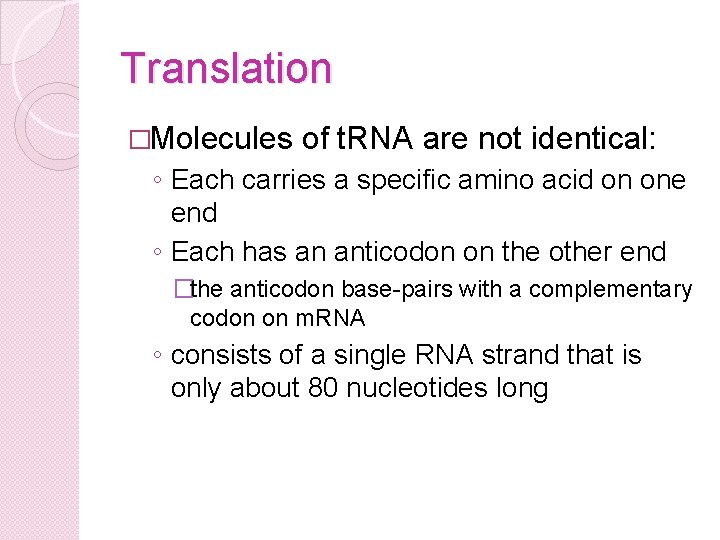
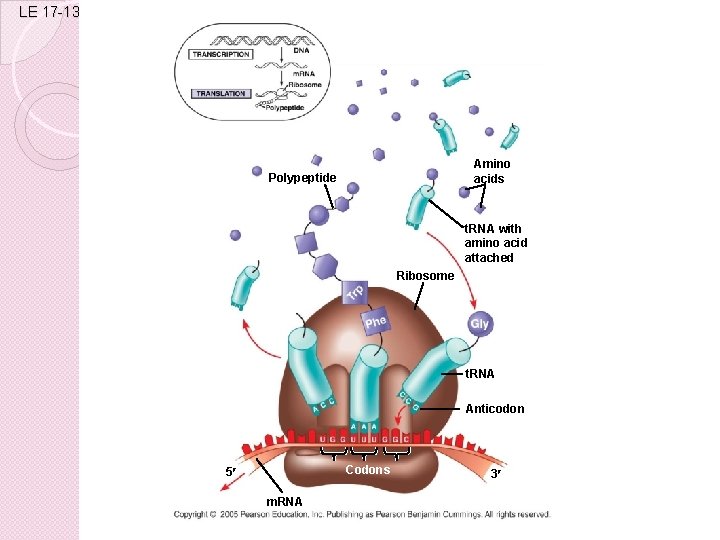
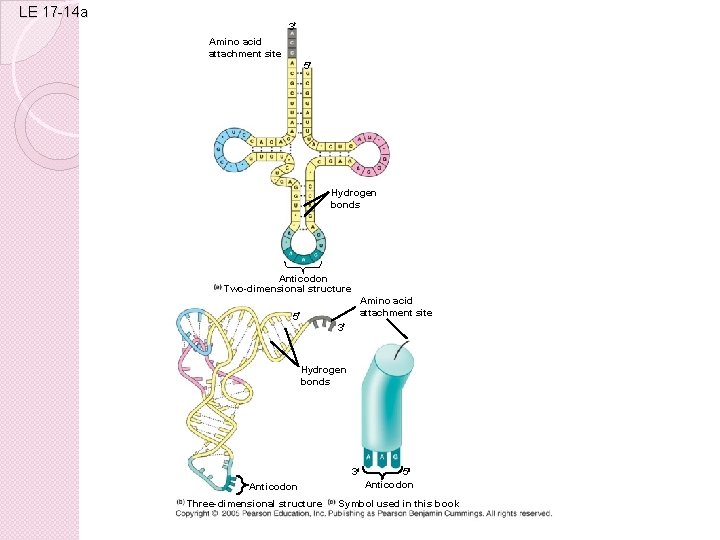
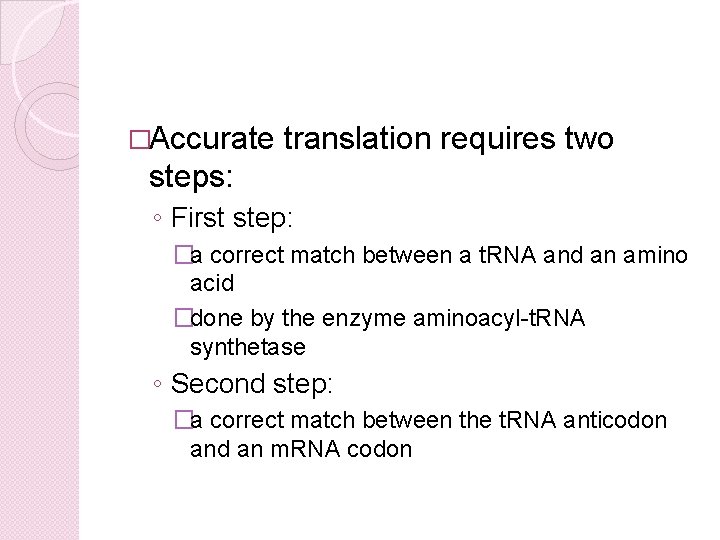
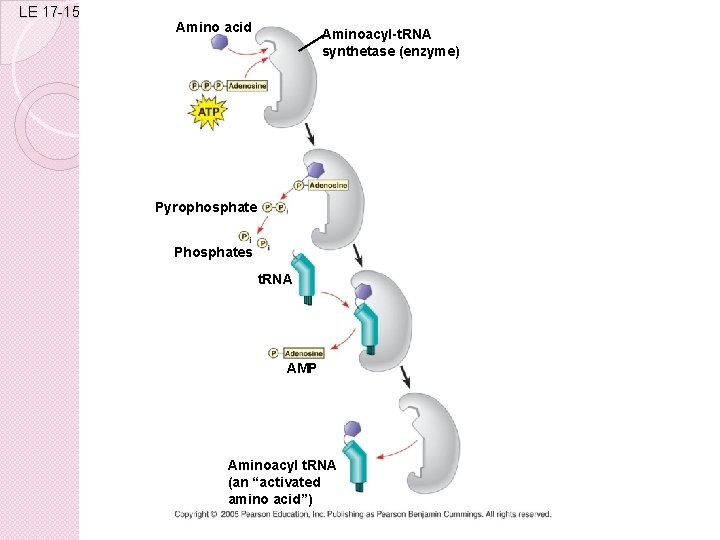
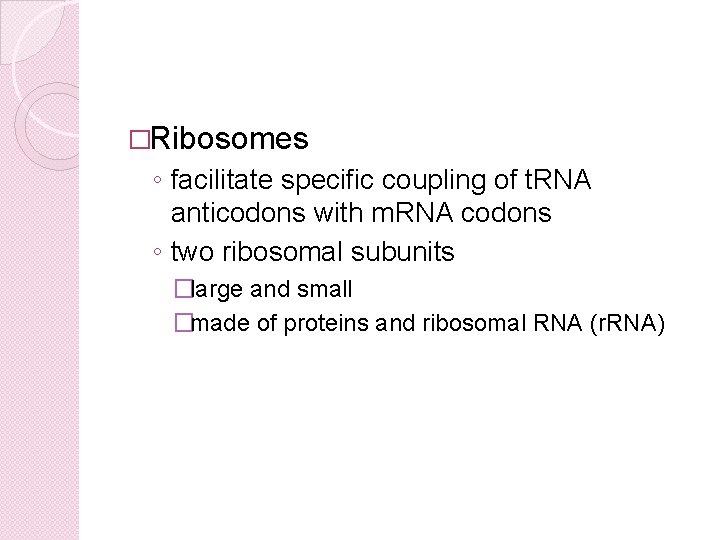
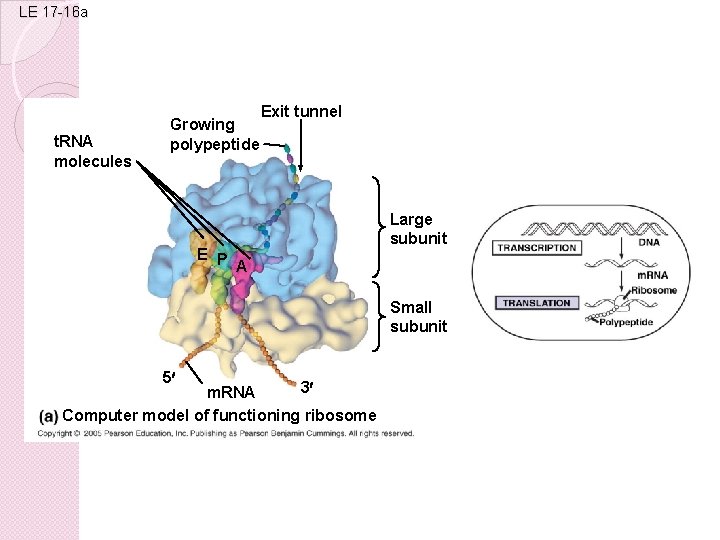
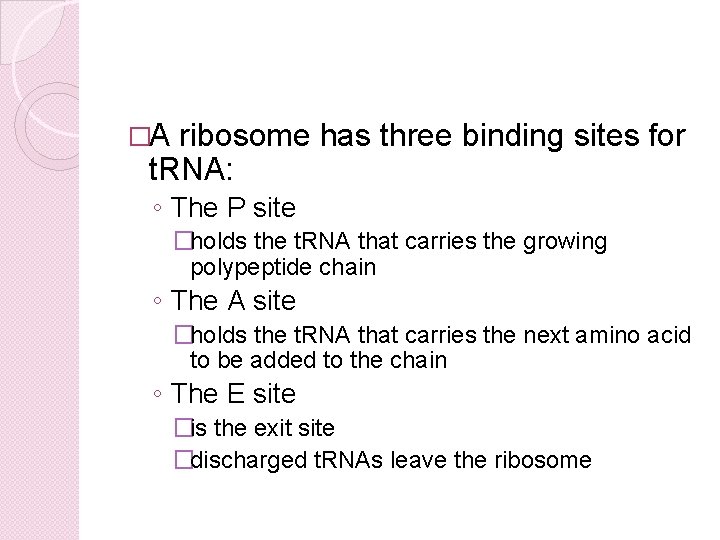
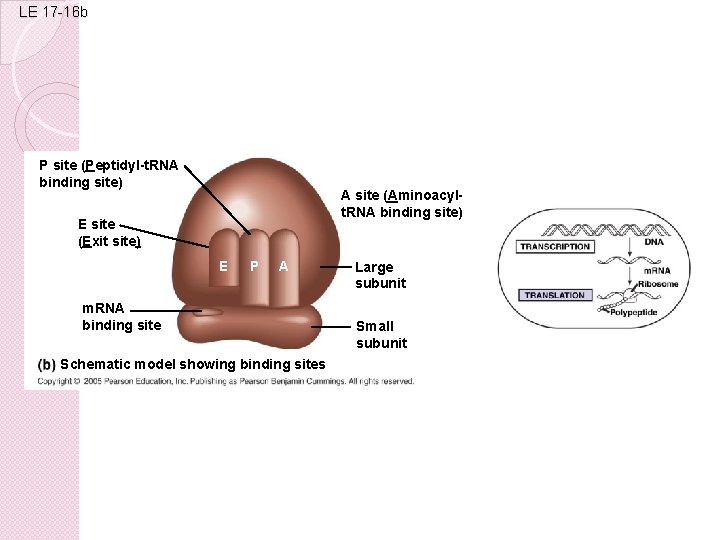
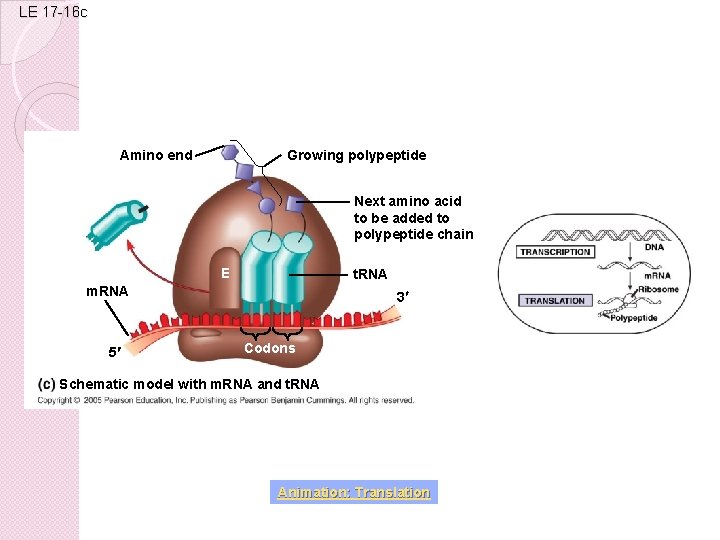
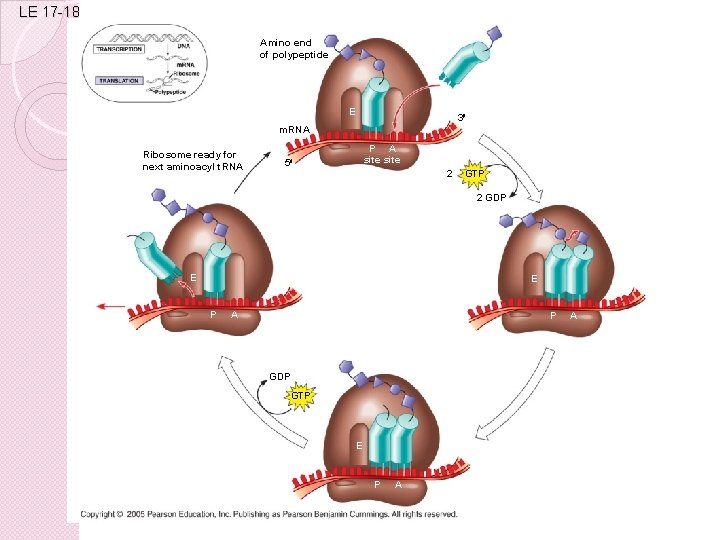
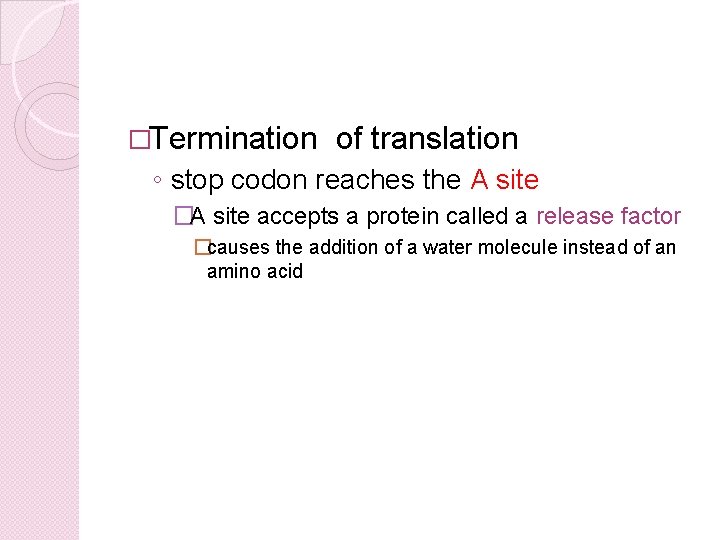
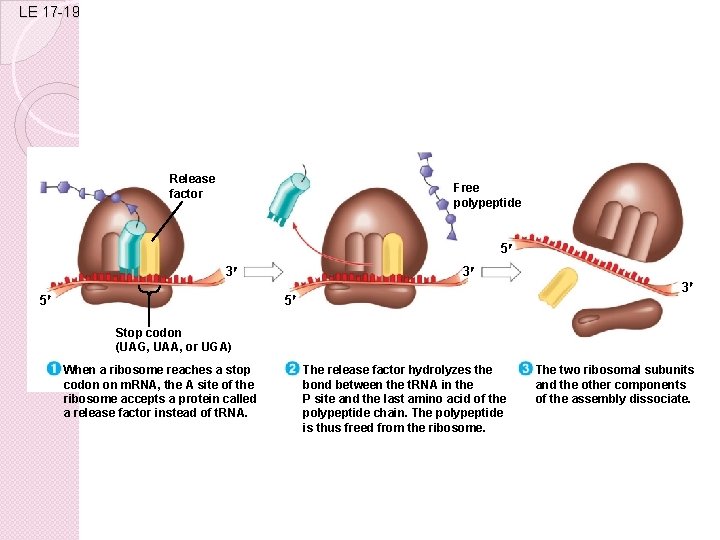
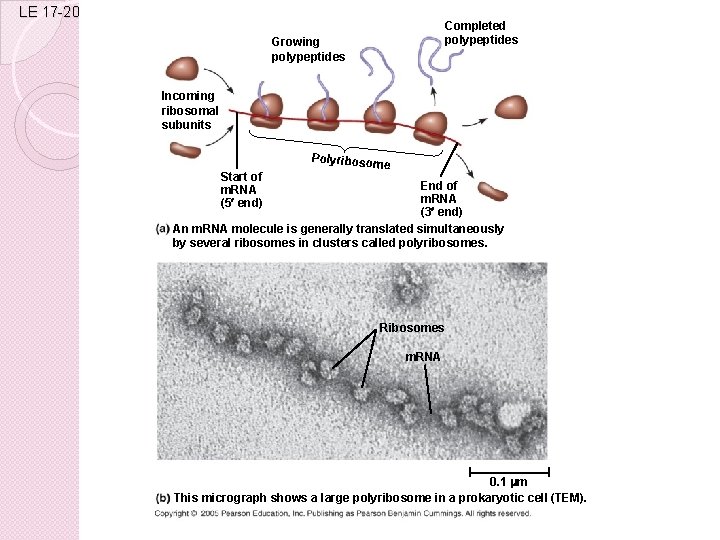
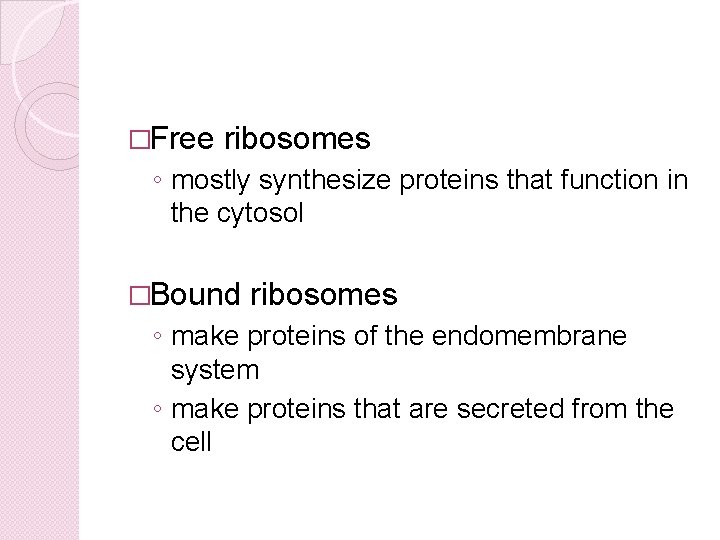
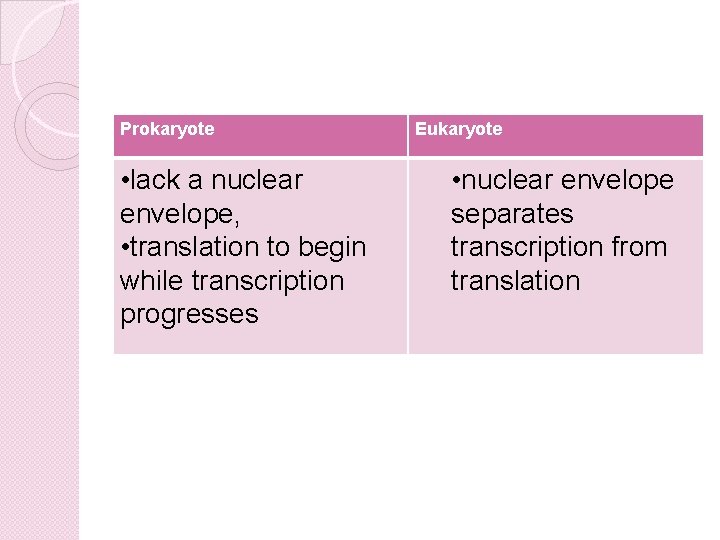
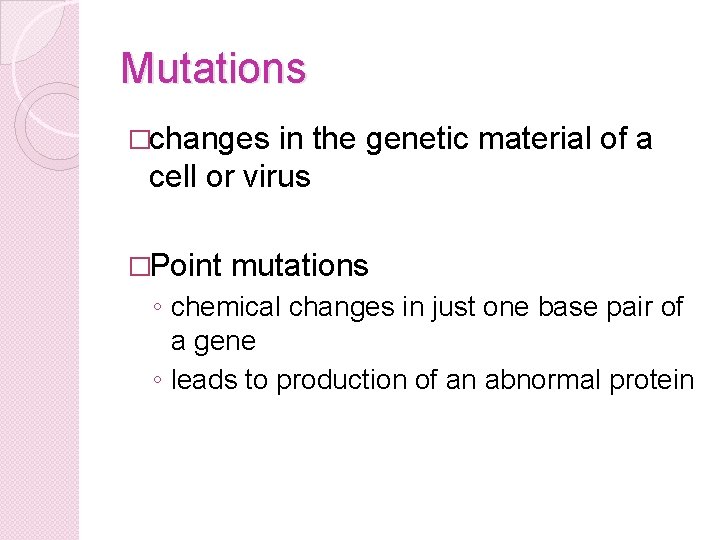
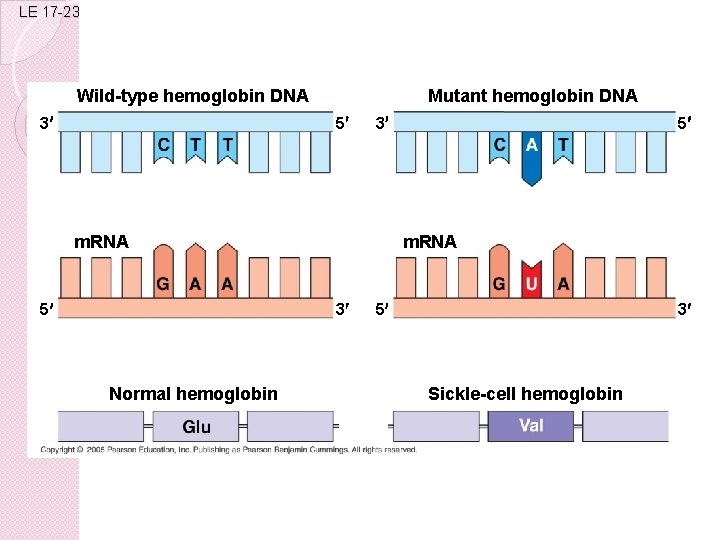
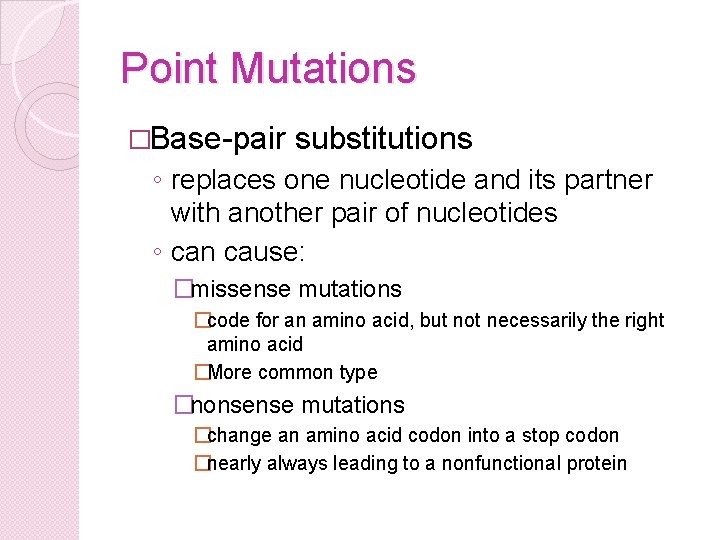
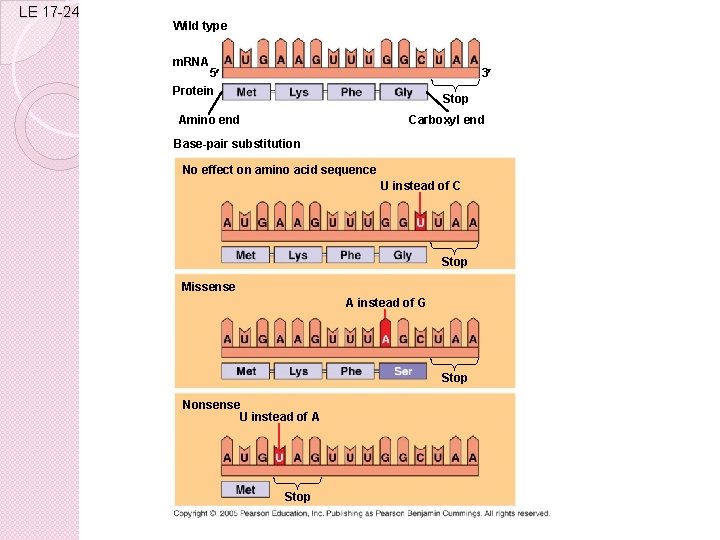
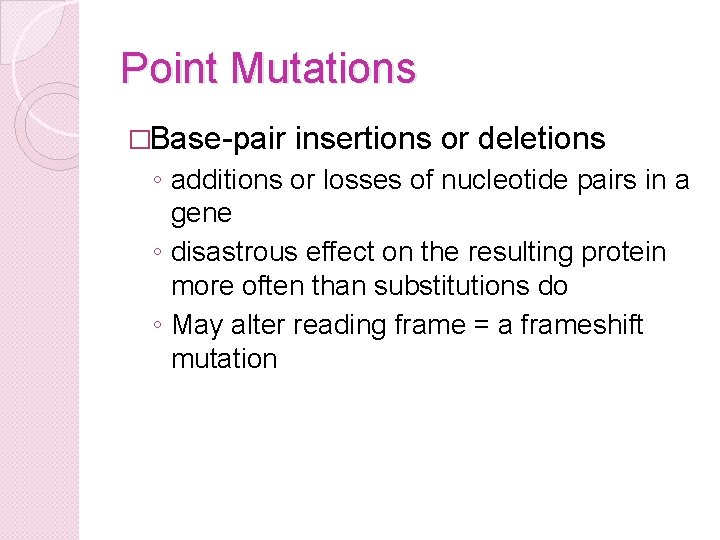
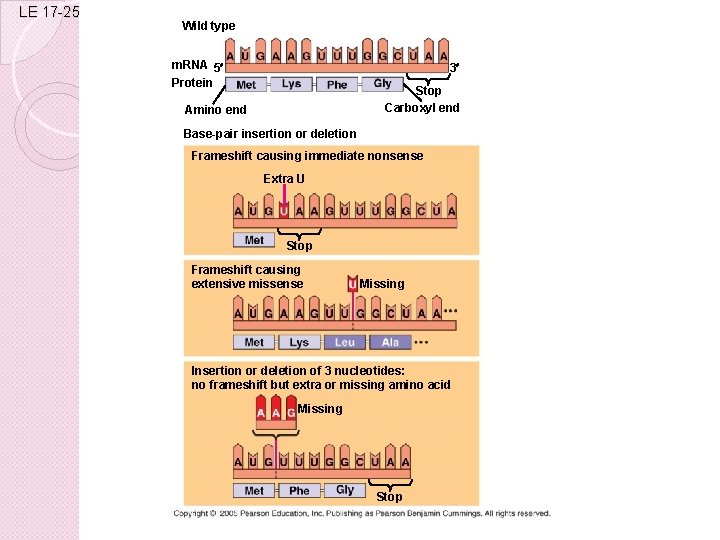
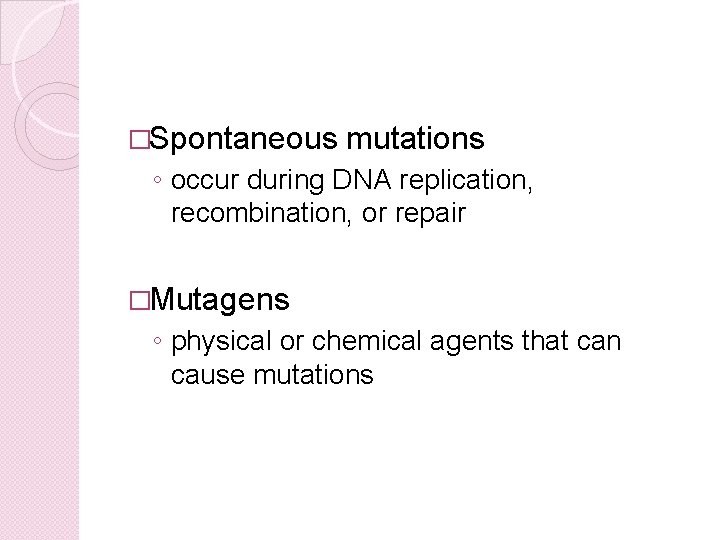
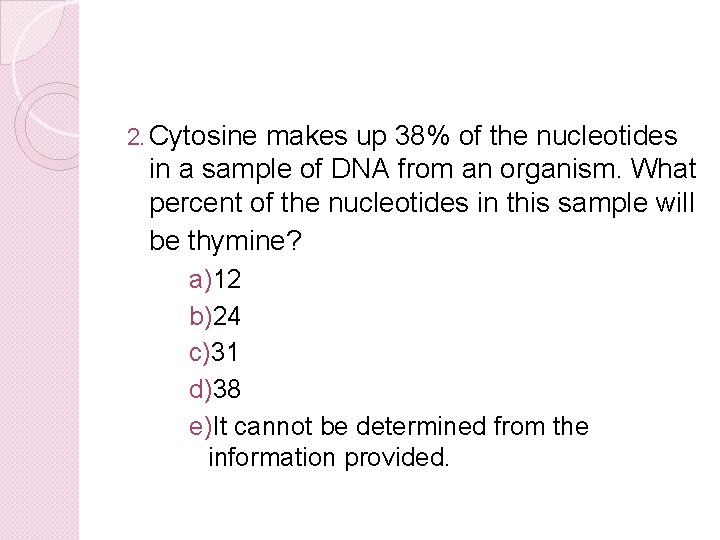
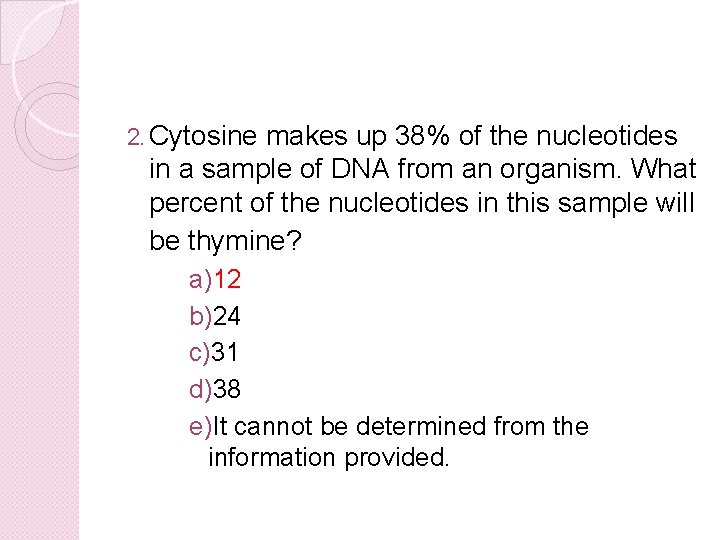
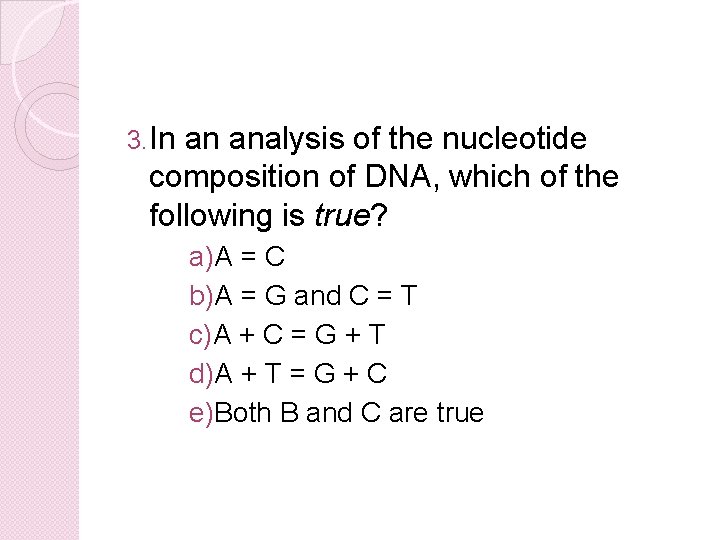
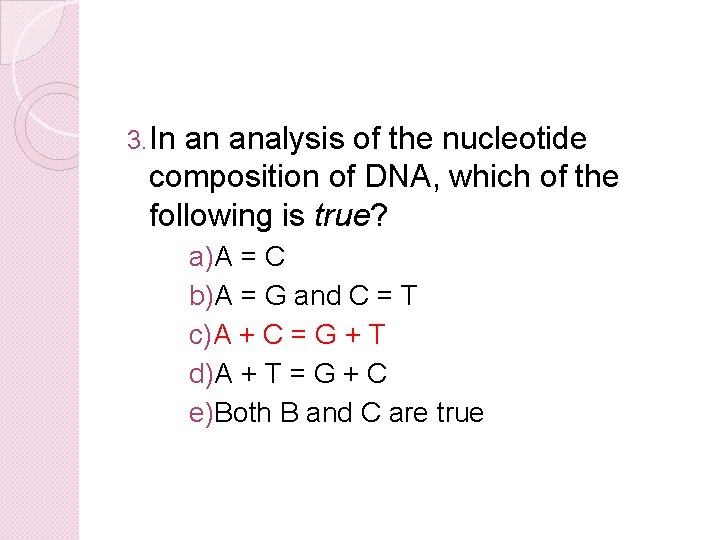
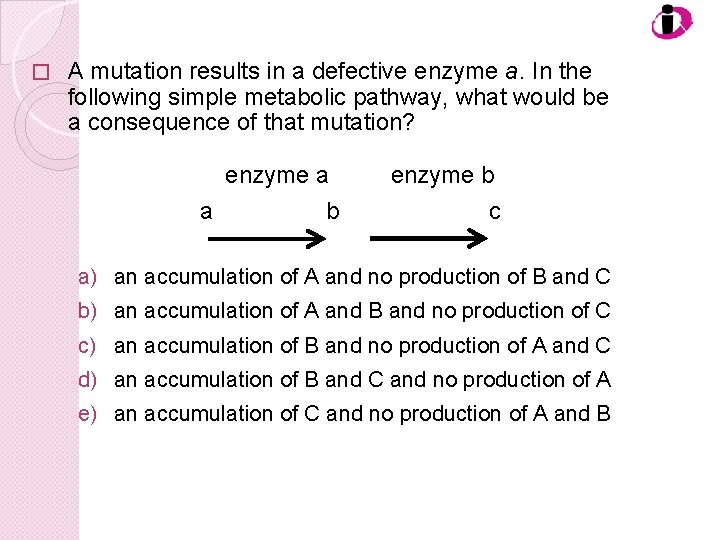
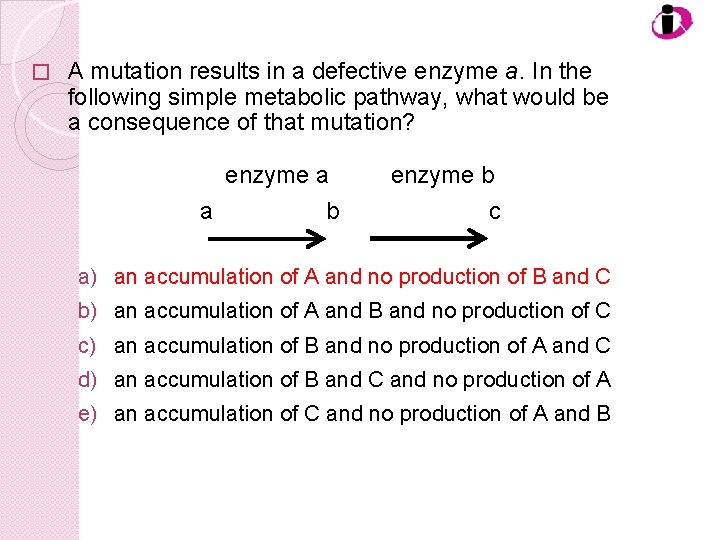
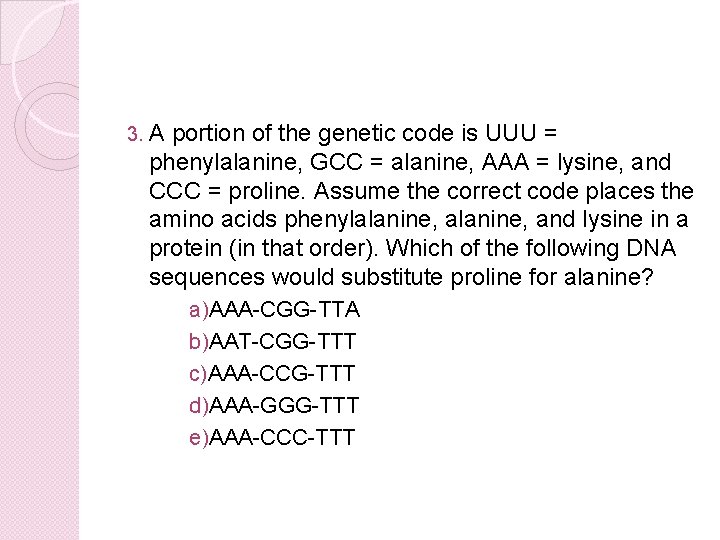
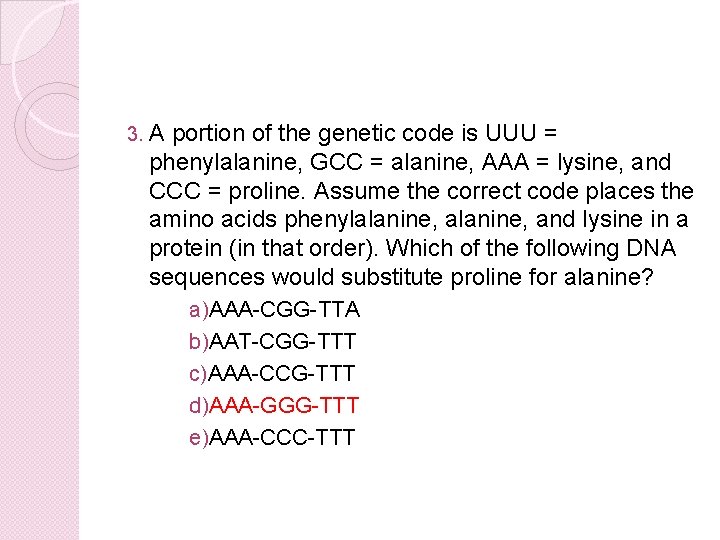
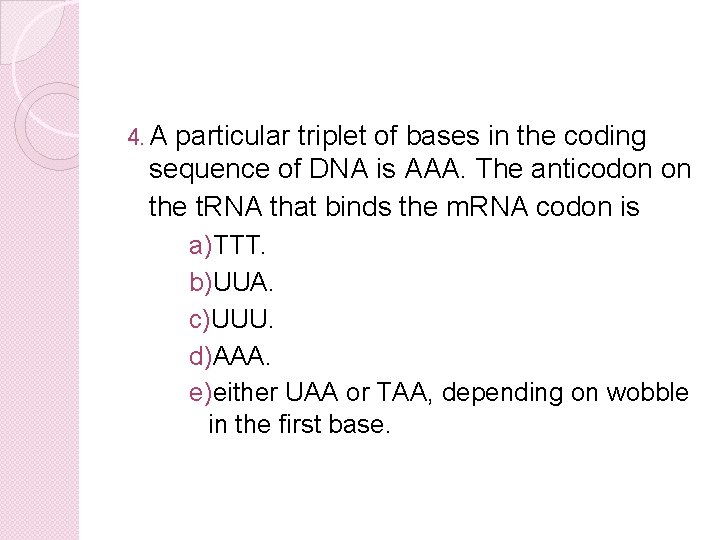
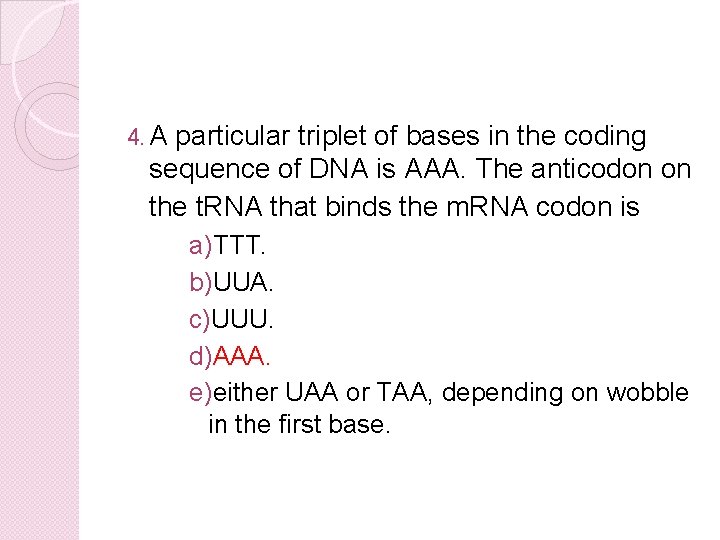
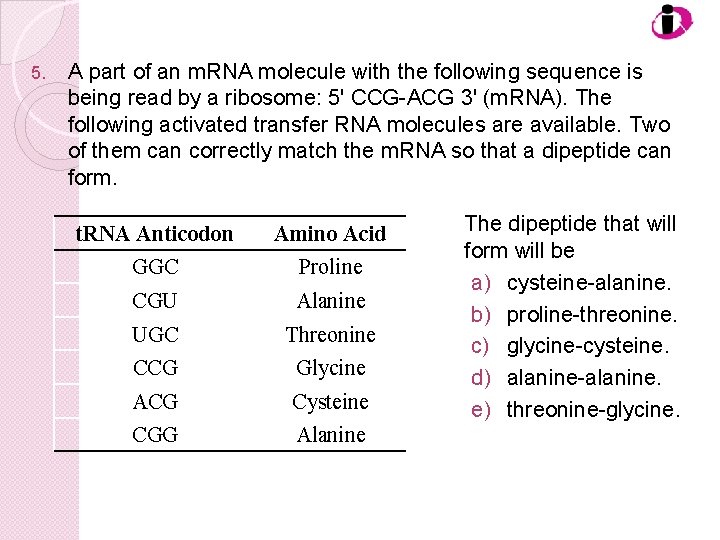
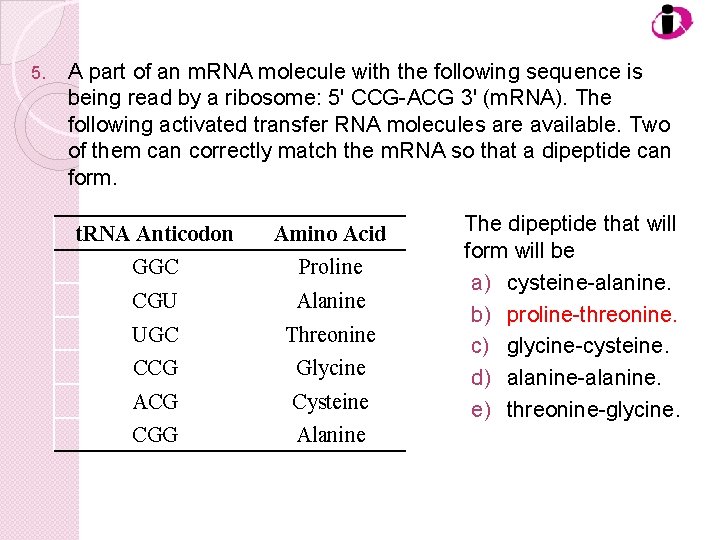
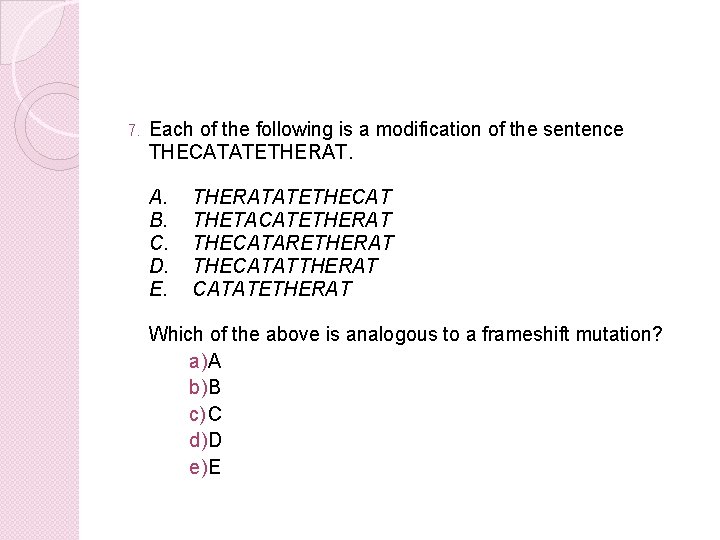
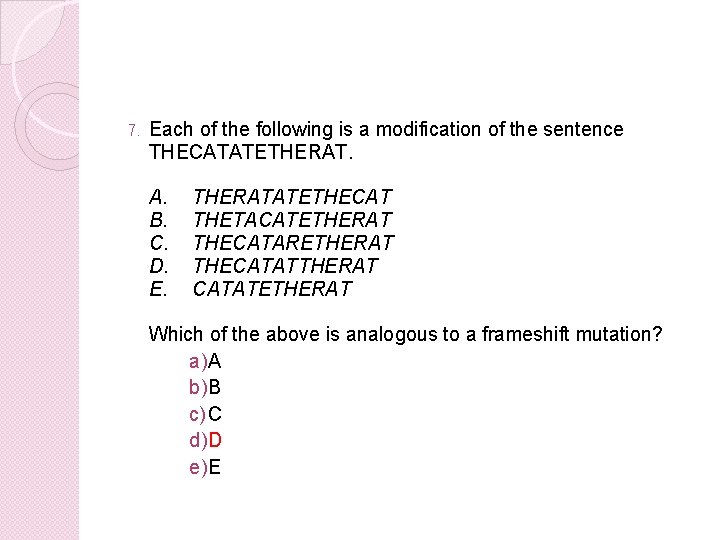
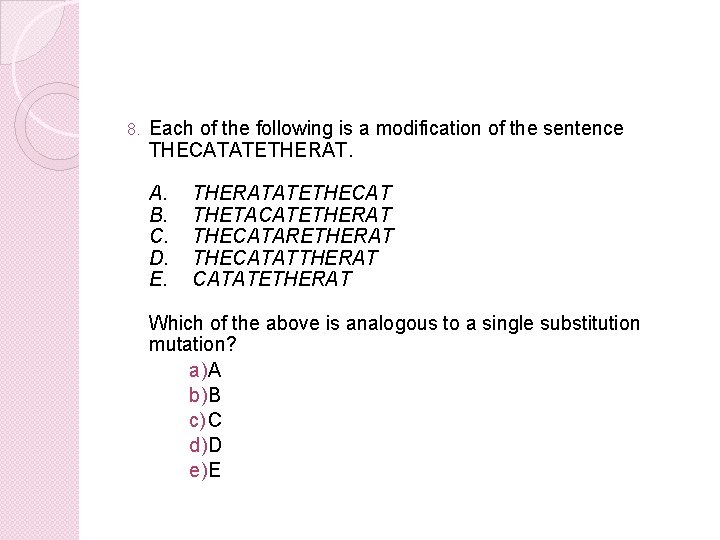
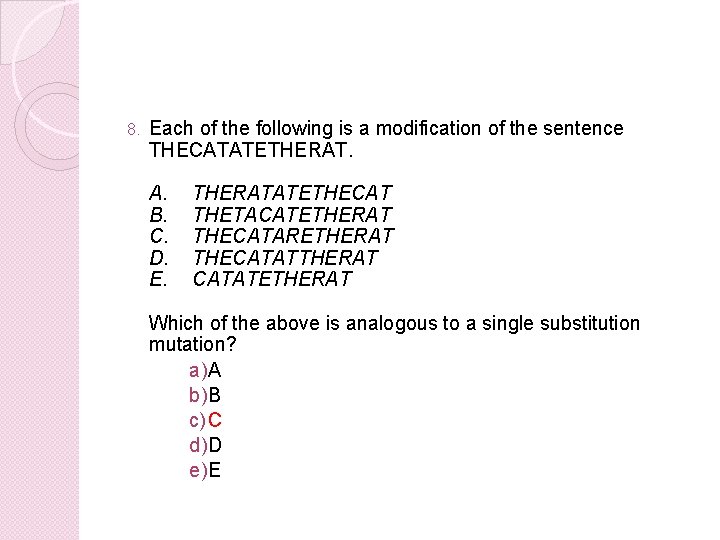
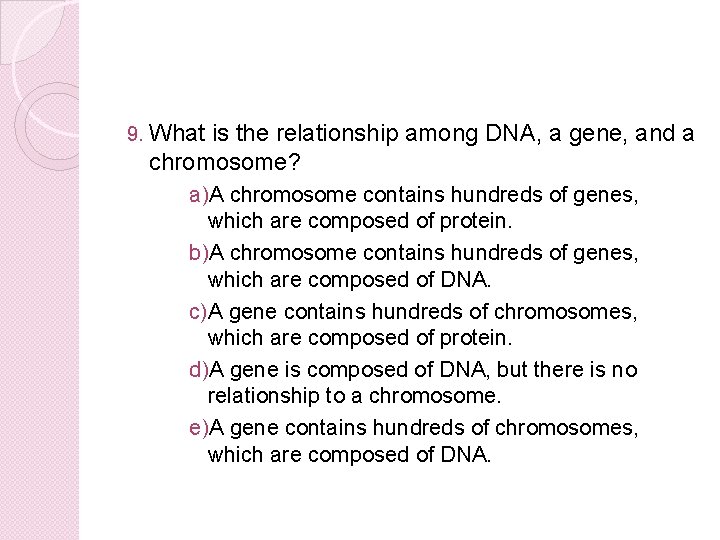
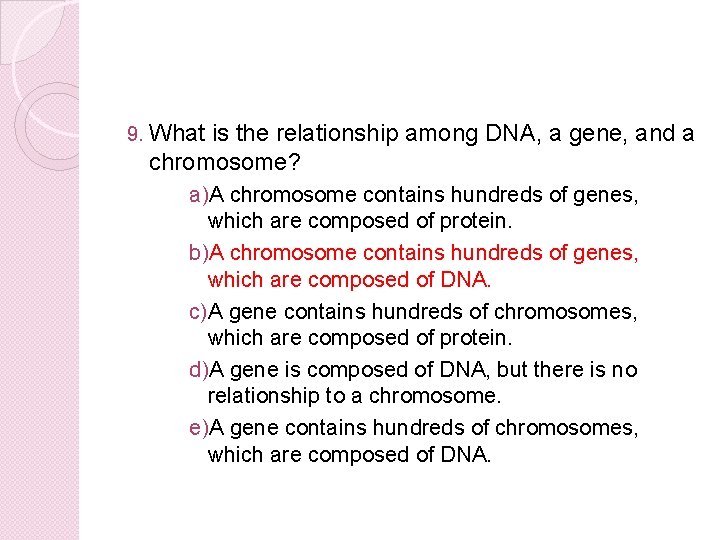
- Slides: 89
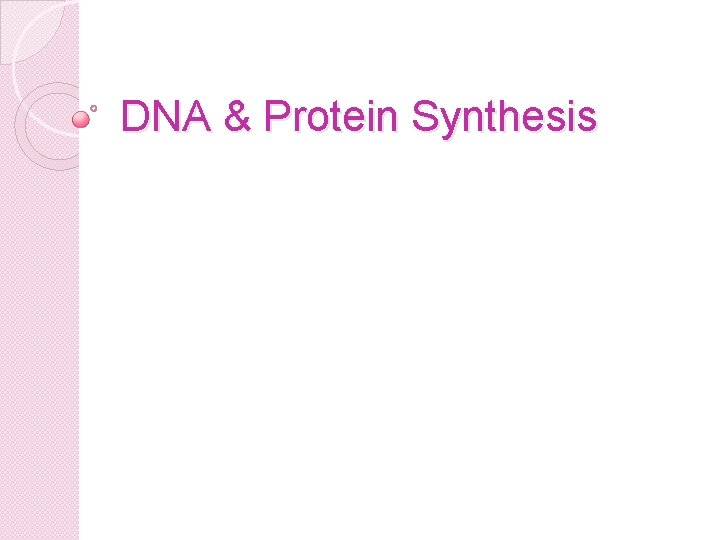
DNA & Protein Synthesis
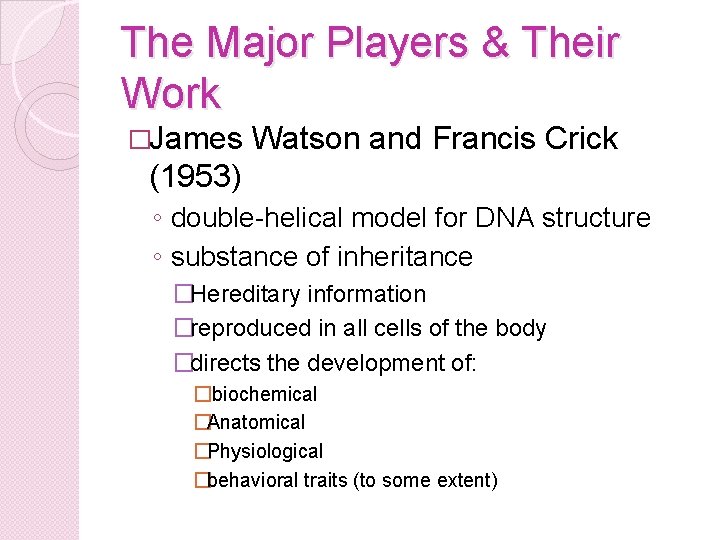
The Major Players & Their Work �James Watson and Francis Crick (1953) ◦ double-helical model for DNA structure ◦ substance of inheritance �Hereditary information �reproduced in all cells of the body �directs the development of: �biochemical �Anatomical �Physiological �behavioral traits (to some extent)
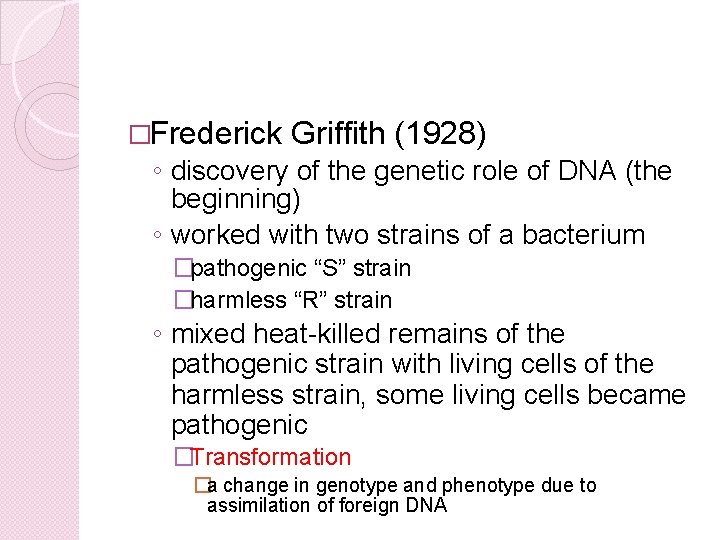
�Frederick Griffith (1928) ◦ discovery of the genetic role of DNA (the beginning) ◦ worked with two strains of a bacterium �pathogenic “S” strain �harmless “R” strain ◦ mixed heat-killed remains of the pathogenic strain with living cells of the harmless strain, some living cells became pathogenic �Transformation �a change in genotype and phenotype due to assimilation of foreign DNA
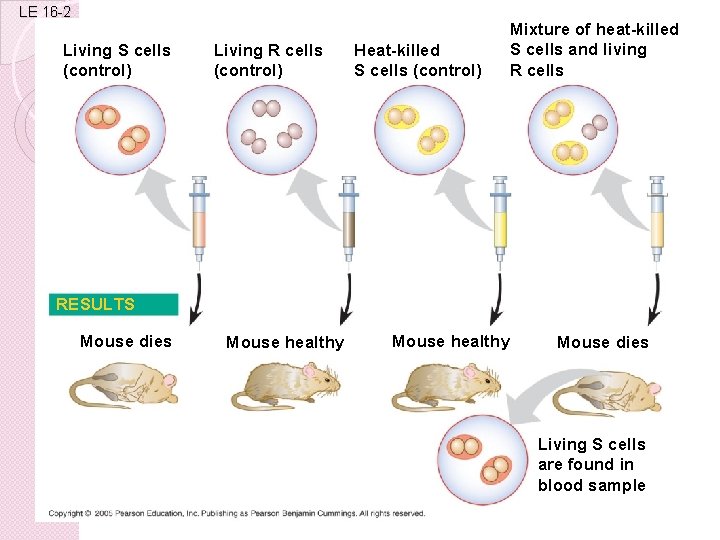
LE 16 -2 Living S cells (control) Living R cells (control) Heat-killed S cells (control) Mixture of heat-killed S cells and living R cells RESULTS Mouse dies Mouse healthy Mouse dies Living S cells are found in blood sample
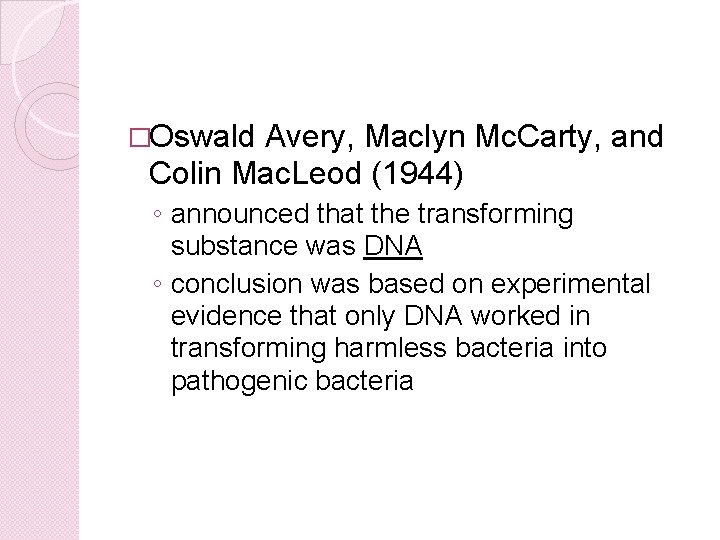
�Oswald Avery, Maclyn Mc. Carty, and Colin Mac. Leod (1944) ◦ announced that the transforming substance was DNA ◦ conclusion was based on experimental evidence that only DNA worked in transforming harmless bacteria into pathogenic bacteria
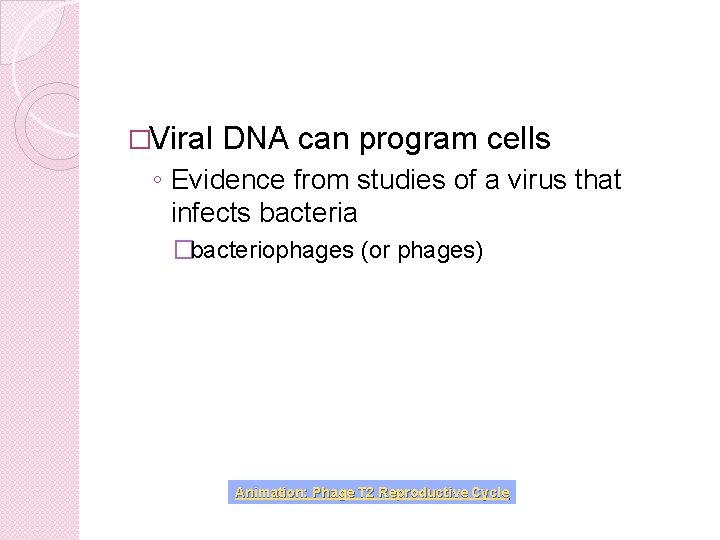
�Viral DNA can program cells ◦ Evidence from studies of a virus that infects bacteria �bacteriophages (or phages) Animation: Phage T 2 Reproductive Cycle
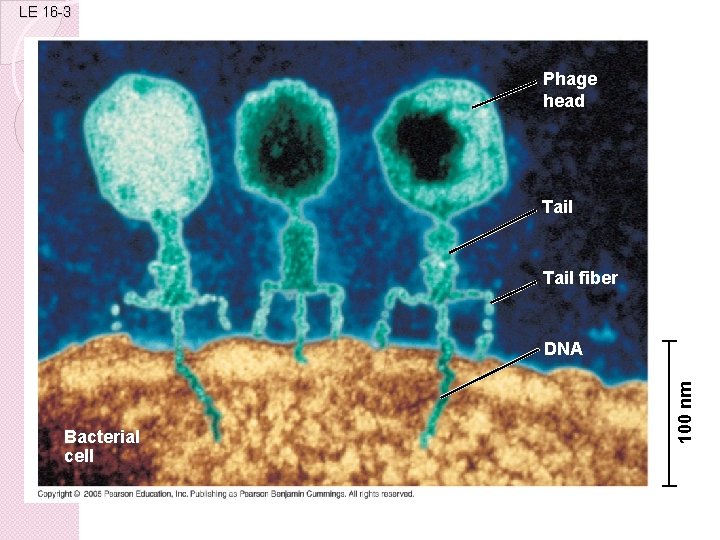
LE 16 -3 Phage head Tail fiber Bacterial cell 100 nm DNA
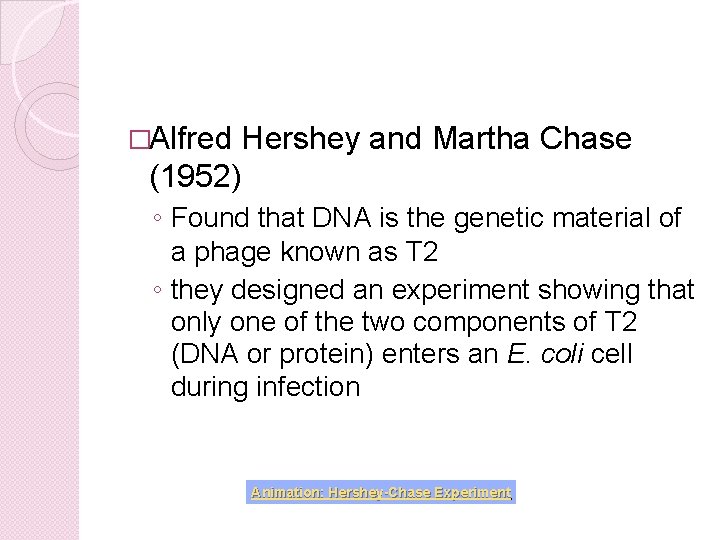
�Alfred Hershey and Martha Chase (1952) ◦ Found that DNA is the genetic material of a phage known as T 2 ◦ they designed an experiment showing that only one of the two components of T 2 (DNA or protein) enters an E. coli cell during infection Animation: Hershey-Chase Experiment
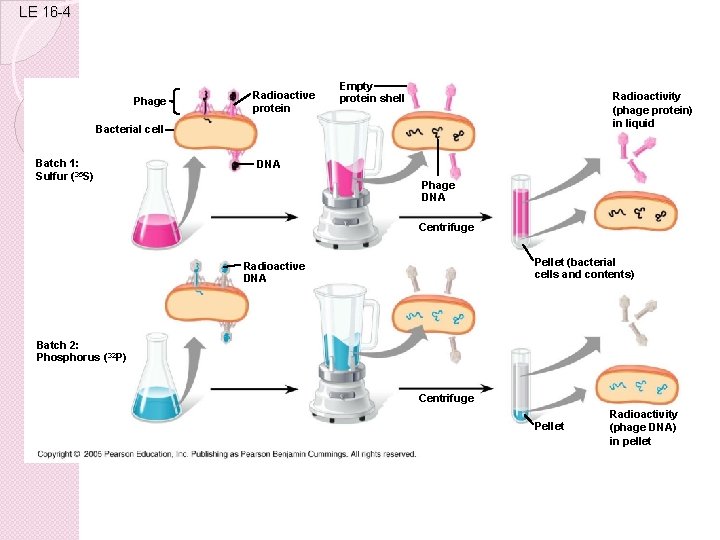
LE 16 -4 Phage Radioactive protein Empty protein shell Radioactivity (phage protein) in liquid Bacterial cell Batch 1: Sulfur (35 S) DNA Phage DNA Centrifuge Pellet (bacterial cells and contents) Radioactive DNA Batch 2: Phosphorus (32 P) Centrifuge Pellet Radioactivity (phage DNA) in pellet
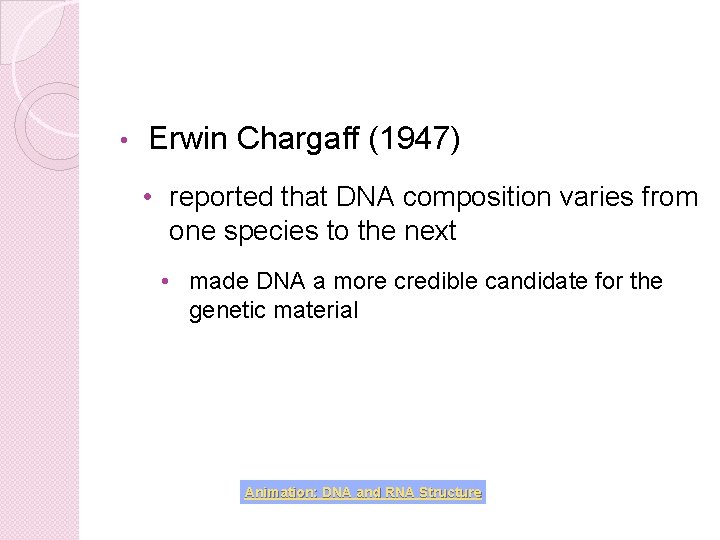
• Erwin Chargaff (1947) • reported that DNA composition varies from one species to the next • made DNA a more credible candidate for the genetic material Animation: DNA and RNA Structure
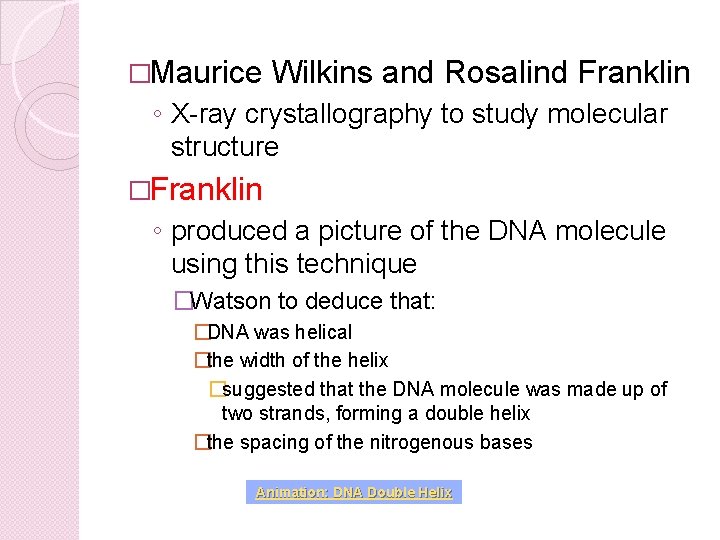
�Maurice Wilkins and Rosalind Franklin ◦ X-ray crystallography to study molecular structure �Franklin ◦ produced a picture of the DNA molecule using this technique �Watson to deduce that: �DNA was helical �the width of the helix �suggested that the DNA molecule was made up of two strands, forming a double helix �the spacing of the nitrogenous bases Animation: DNA Double Helix
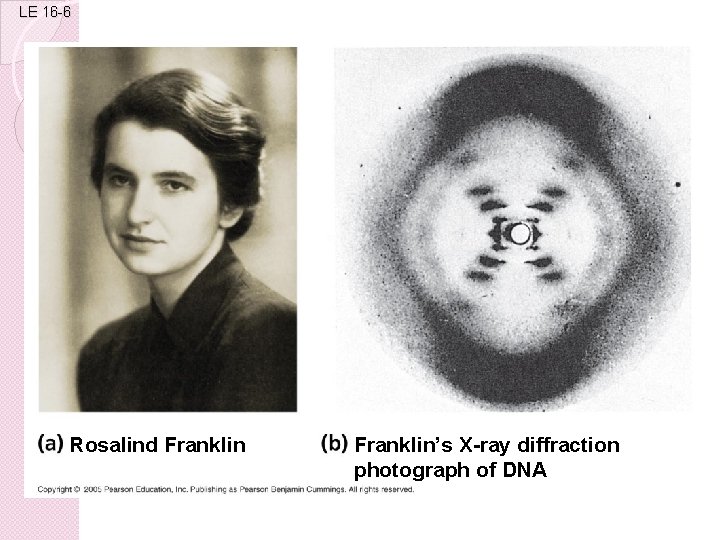
LE 16 -6 Rosalind Franklin’s X-ray diffraction photograph of DNA
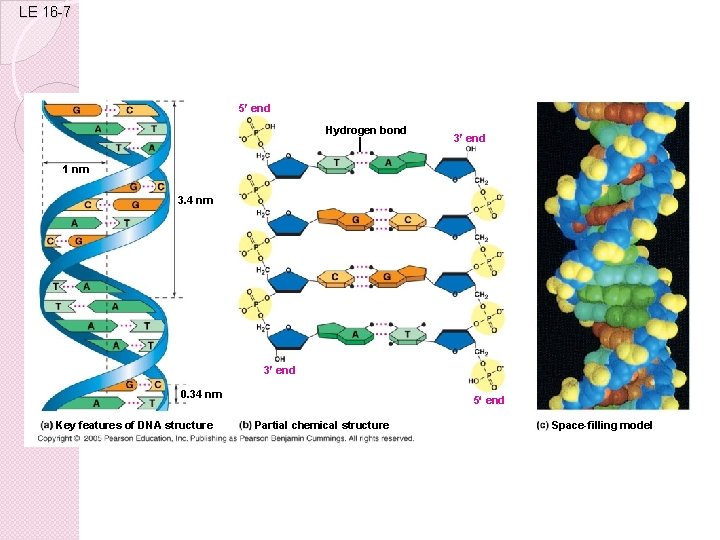
LE 16 -7 5 end Hydrogen bond 3 end 1 nm 3. 4 nm 3 end 0. 34 nm Key features of DNA structure 5 end Partial chemical structure Space-filling model
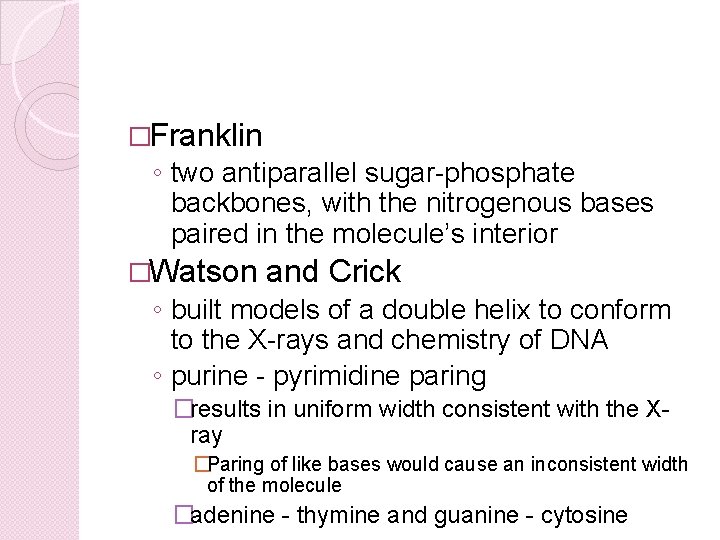
�Franklin ◦ two antiparallel sugar-phosphate backbones, with the nitrogenous bases paired in the molecule’s interior �Watson and Crick ◦ built models of a double helix to conform to the X-rays and chemistry of DNA ◦ purine - pyrimidine paring �results in uniform width consistent with the Xray �Paring of like bases would cause an inconsistent width of the molecule �adenine - thymine and guanine - cytosine
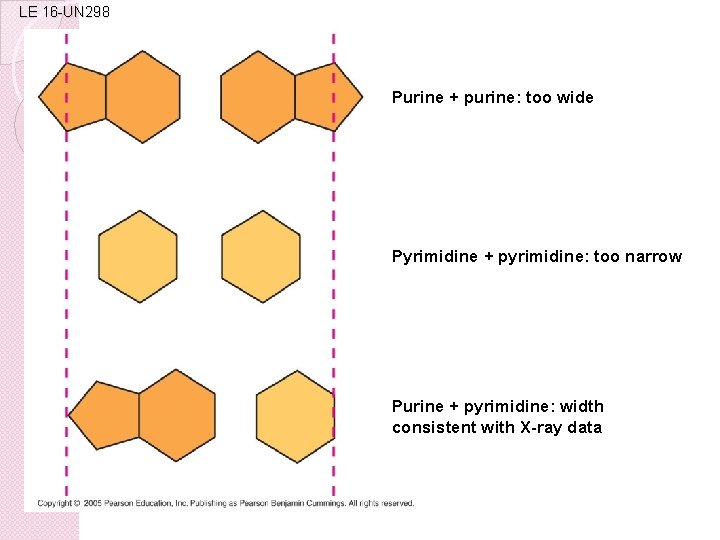
LE 16 -UN 298 Purine + purine: too wide Pyrimidine + pyrimidine: too narrow Purine + pyrimidine: width consistent with X-ray data
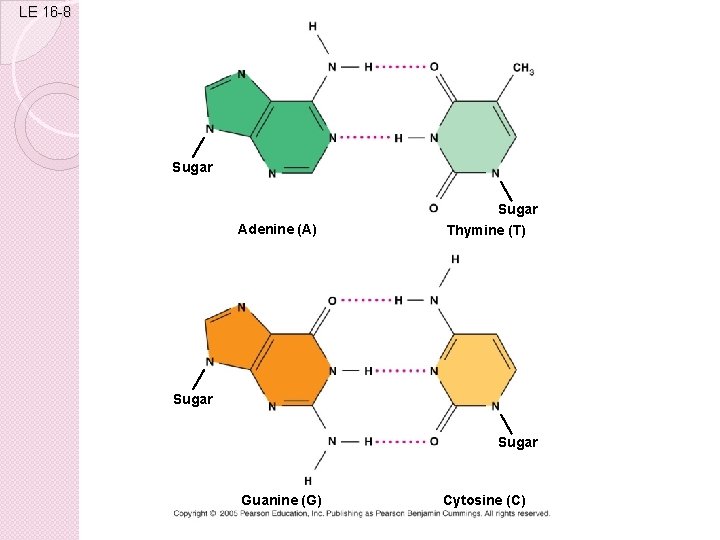
LE 16 -8 Sugar Adenine (A) Sugar Thymine (T) Sugar Guanine (G) Cytosine (C)
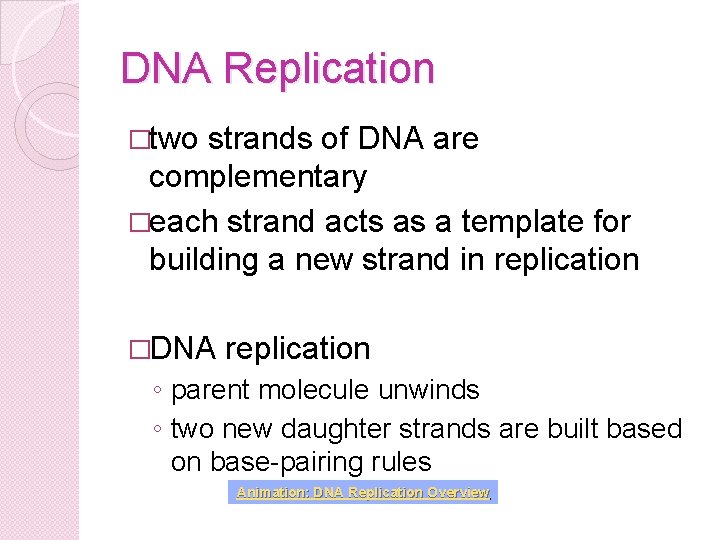
DNA Replication �two strands of DNA are complementary �each strand acts as a template for building a new strand in replication �DNA replication ◦ parent molecule unwinds ◦ two new daughter strands are built based on base-pairing rules Animation: DNA Replication Overview
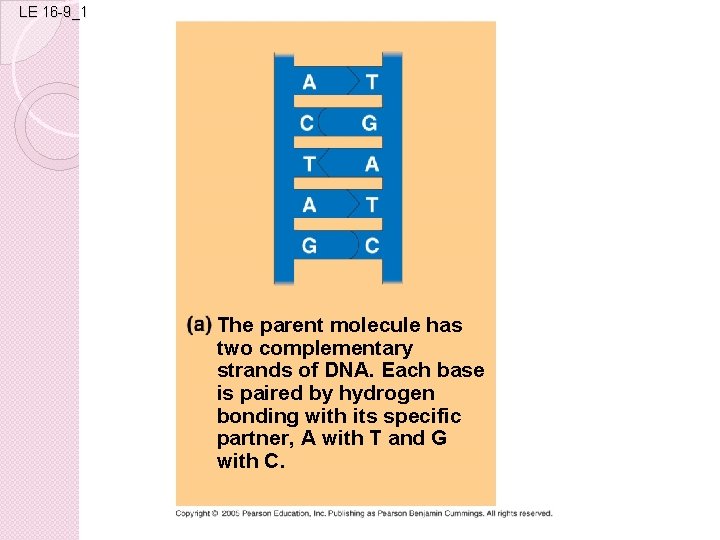
LE 16 -9_1 The parent molecule has two complementary strands of DNA. Each base is paired by hydrogen bonding with its specific partner, A with T and G with C.
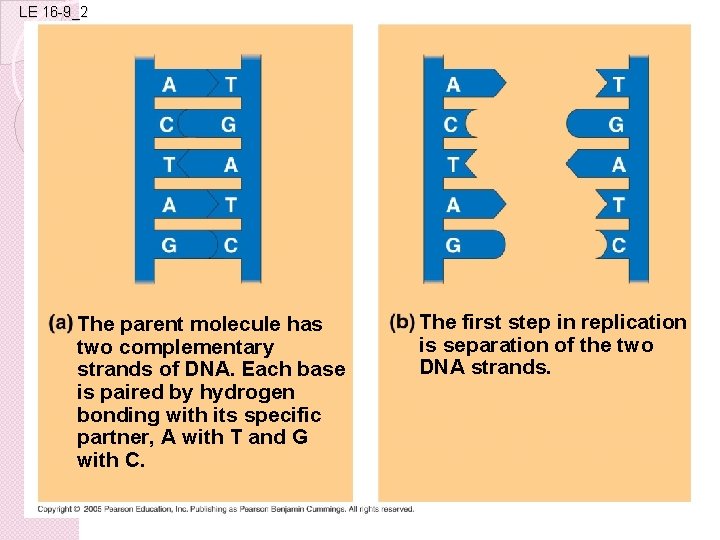
LE 16 -9_2 The parent molecule has two complementary strands of DNA. Each base is paired by hydrogen bonding with its specific partner, A with T and G with C. The first step in replication is separation of the two DNA strands.
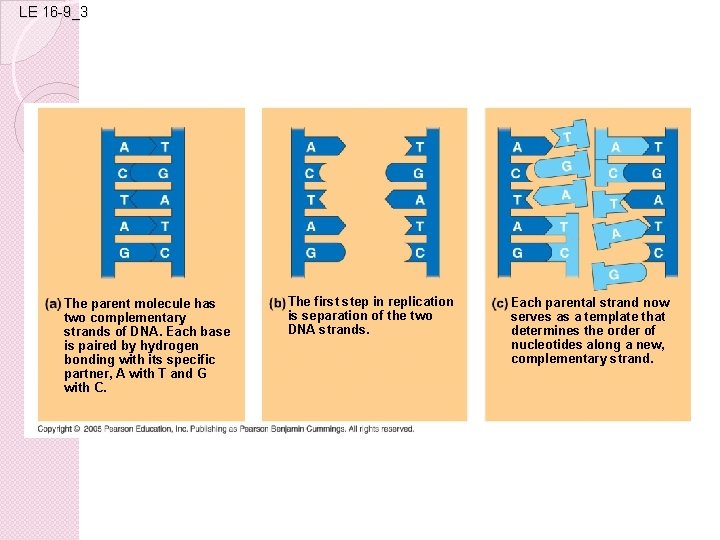
LE 16 -9_3 The parent molecule has two complementary strands of DNA. Each base is paired by hydrogen bonding with its specific partner, A with T and G with C. The first step in replication is separation of the two DNA strands. Each parental strand now serves as a template that determines the order of nucleotides along a new, complementary strand.
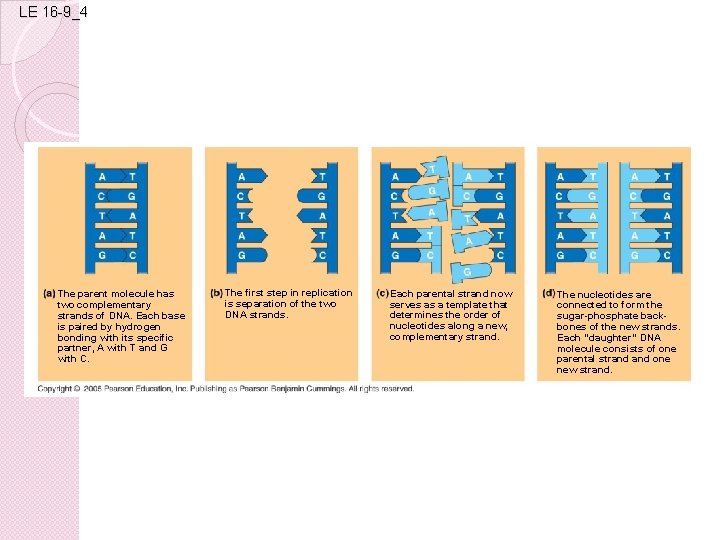
LE 16 -9_4 The parent molecule has two complementary strands of DNA. Each base is paired by hydrogen bonding with its specific partner, A with T and G with C. The first step in replication is separation of the two DNA strands. Each parental strand now serves as a template that determines the order of nucleotides along a new, complementary strand. The nucleotides are connected to form the sugar-phosphate backbones of the new strands. Each “daughter” DNA molecule consists of one parental strand one new strand.
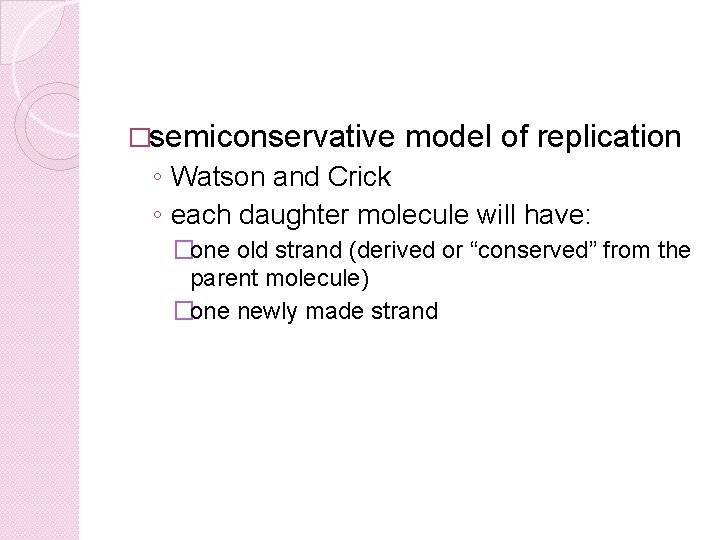
�semiconservative model of replication ◦ Watson and Crick ◦ each daughter molecule will have: �one old strand (derived or “conserved” from the parent molecule) �one newly made strand
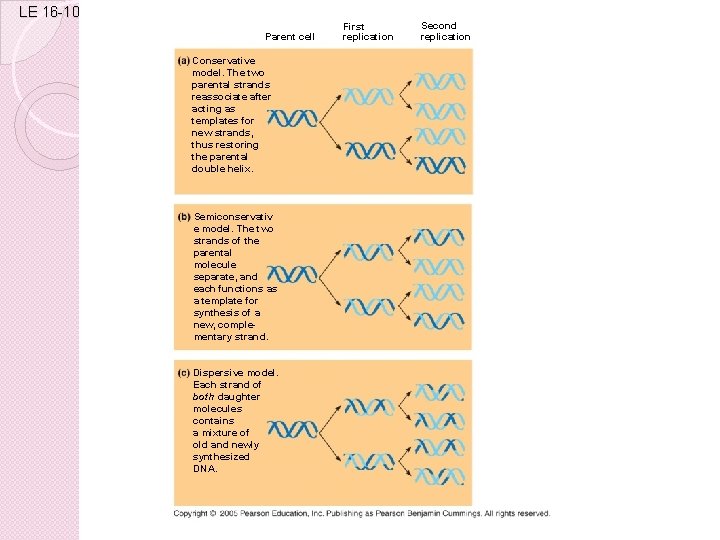
LE 16 -10 Parent cell Conservative model. The two parental strands reassociate after acting as templates for new strands, thus restoring the parental double helix. Semiconservativ e model. The two strands of the parental molecule separate, and each functions as a template for synthesis of a new, complementary strand. Dispersive model. Each strand of both daughter molecules contains a mixture of old and newly synthesized DNA. First replication Second replication
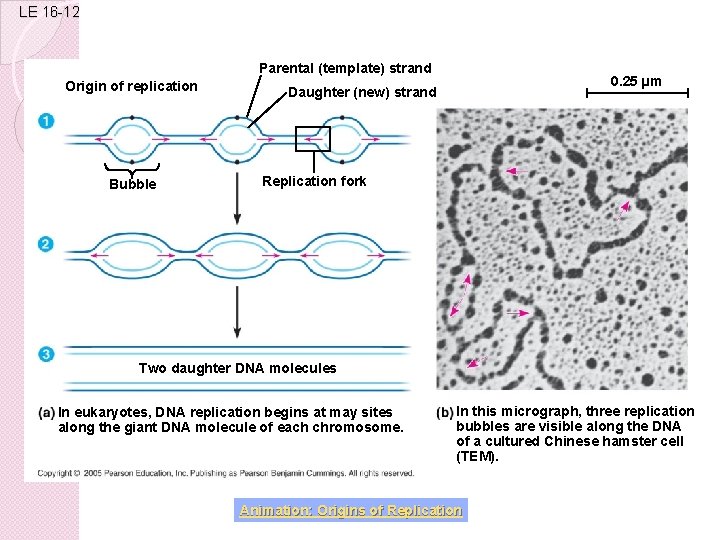
LE 16 -12 Parental (template) strand Origin of replication Bubble 0. 25 µm Daughter (new) strand Replication fork Two daughter DNA molecules In eukaryotes, DNA replication begins at may sites along the giant DNA molecule of each chromosome. In this micrograph, three replication bubbles are visible along the DNA of a cultured Chinese hamster cell (TEM). Animation: Origins of Replication
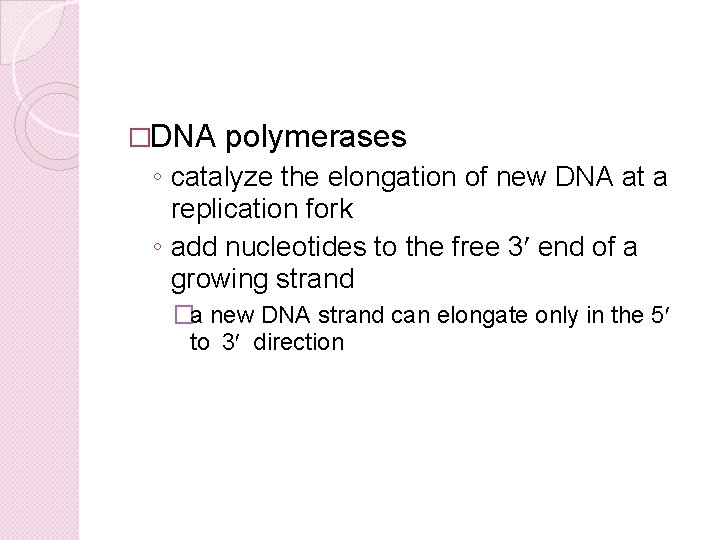
�DNA polymerases ◦ catalyze the elongation of new DNA at a replication fork ◦ add nucleotides to the free 3 end of a growing strand �a new DNA strand can elongate only in the 5 to 3 direction
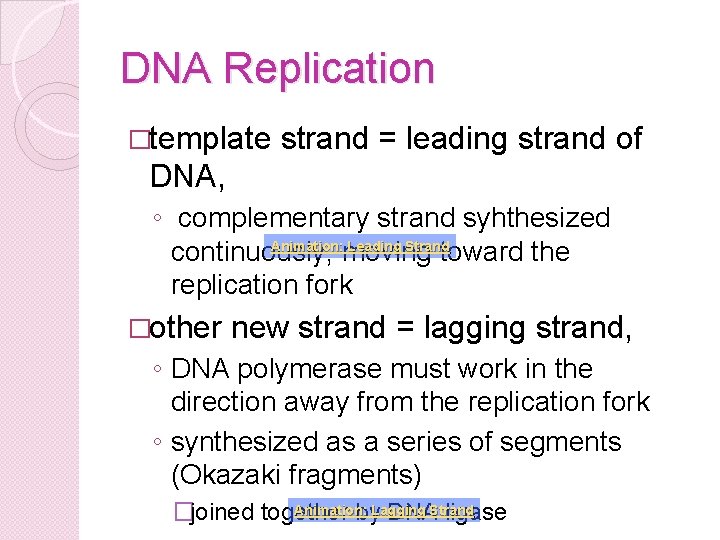
DNA Replication �template strand = leading strand of DNA, ◦ complementary strand syhthesized Animation: Leading Strand continuously, moving toward the replication fork �other new strand = lagging strand, ◦ DNA polymerase must work in the direction away from the replication fork ◦ synthesized as a series of segments (Okazaki fragments) Animation : Lagging �joined together by DNAStrand ligase
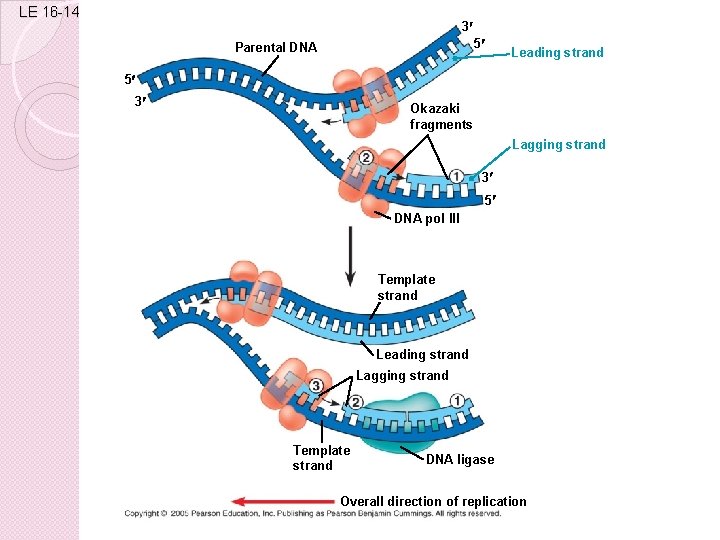
LE 16 -14 3 5 Parental DNA Leading strand 5 3 Okazaki fragments Lagging strand 3 5 DNA pol III Template strand Leading strand Lagging strand Template strand DNA ligase Overall direction of replication
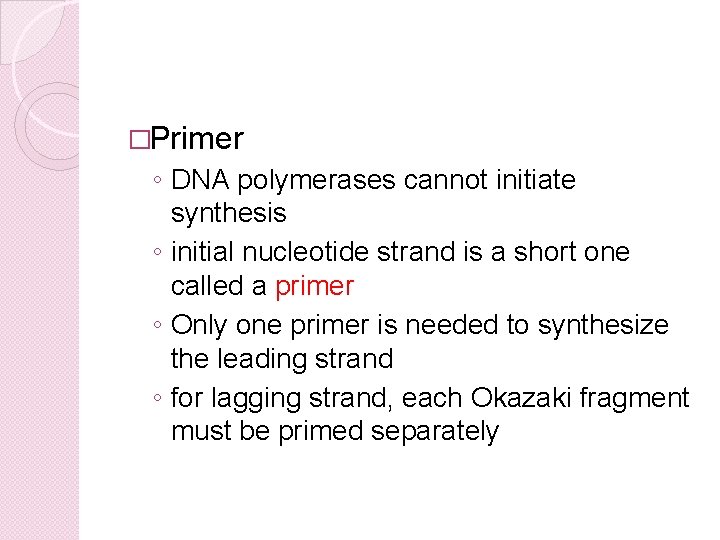
�Primer ◦ DNA polymerases cannot initiate synthesis ◦ initial nucleotide strand is a short one called a primer ◦ Only one primer is needed to synthesize the leading strand ◦ for lagging strand, each Okazaki fragment must be primed separately
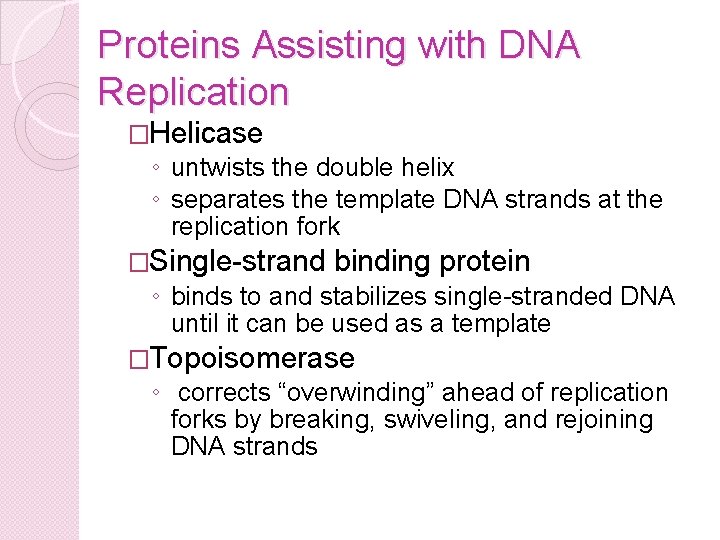
Proteins Assisting with DNA Replication �Helicase ◦ untwists the double helix ◦ separates the template DNA strands at the replication fork �Single-strand binding protein ◦ binds to and stabilizes single-stranded DNA until it can be used as a template �Topoisomerase ◦ corrects “overwinding” ahead of replication forks by breaking, swiveling, and rejoining DNA strands
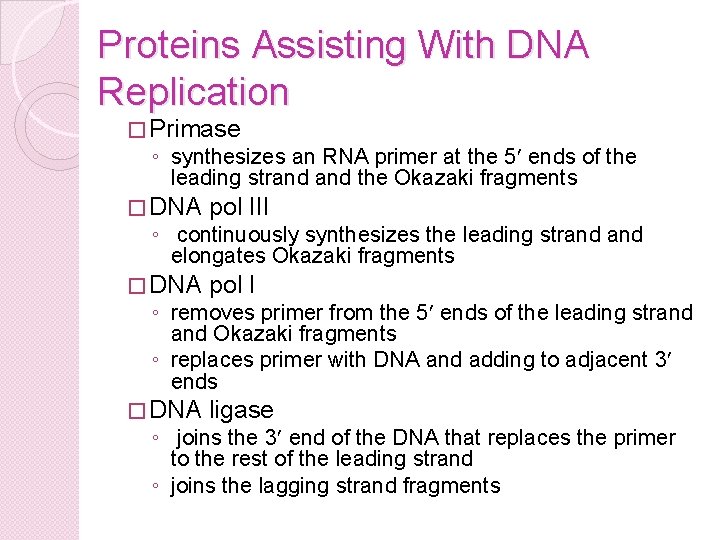
Proteins Assisting With DNA Replication � Primase ◦ synthesizes an RNA primer at the 5 ends of the leading strand the Okazaki fragments � DNA pol III � DNA pol I � DNA ligase ◦ continuously synthesizes the leading strand elongates Okazaki fragments ◦ removes primer from the 5 ends of the leading strand Okazaki fragments ◦ replaces primer with DNA and adding to adjacent 3 ends ◦ joins the 3 end of the DNA that replaces the primer to the rest of the leading strand ◦ joins the lagging strand fragments
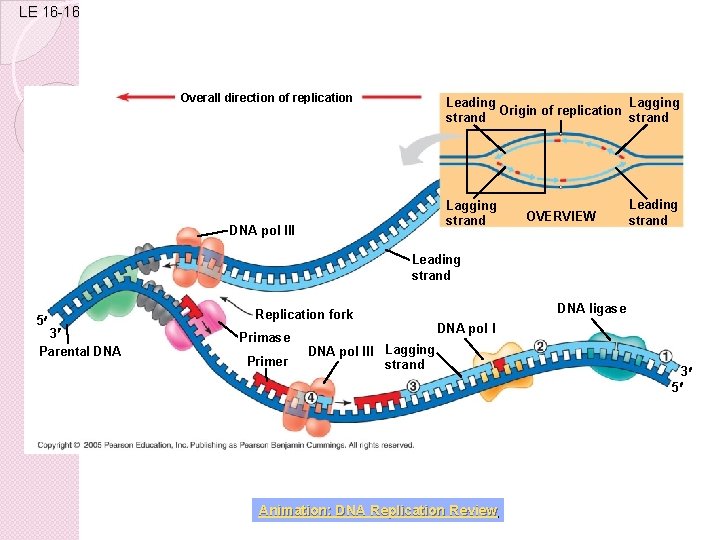
LE 16 -16 Overall direction of replication Lagging Leading Origin of replication strand Lagging strand DNA pol III OVERVIEW Leading strand 5 3 Parental DNA Replication fork Primase Primer DNA ligase DNA pol III Lagging strand Animation: DNA Replication Review 3 5
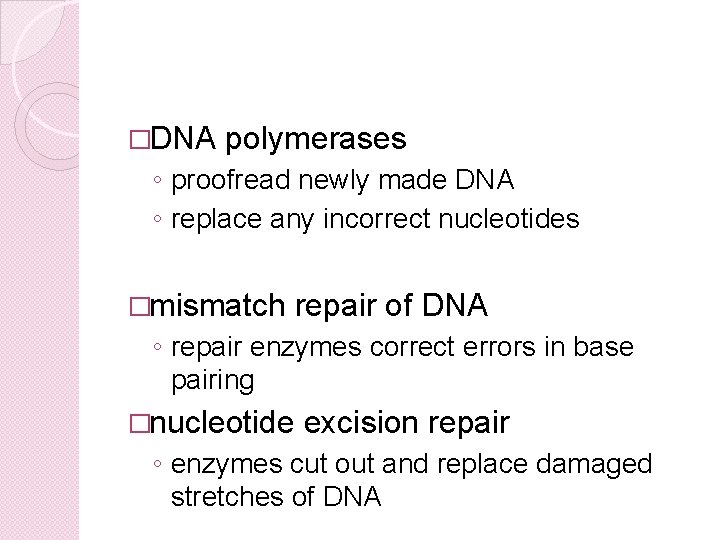
�DNA polymerases ◦ proofread newly made DNA ◦ replace any incorrect nucleotides �mismatch repair of DNA ◦ repair enzymes correct errors in base pairing �nucleotide excision repair ◦ enzymes cut out and replace damaged stretches of DNA
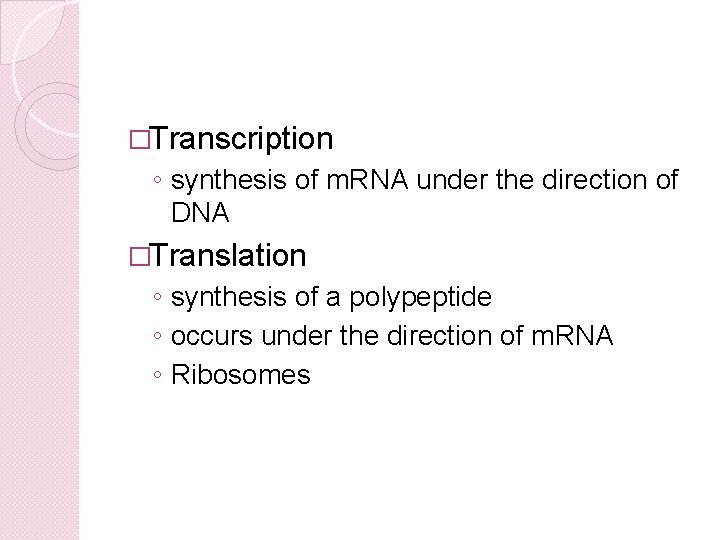
�Transcription ◦ synthesis of m. RNA under the direction of DNA �Translation ◦ synthesis of a polypeptide ◦ occurs under the direction of m. RNA ◦ Ribosomes
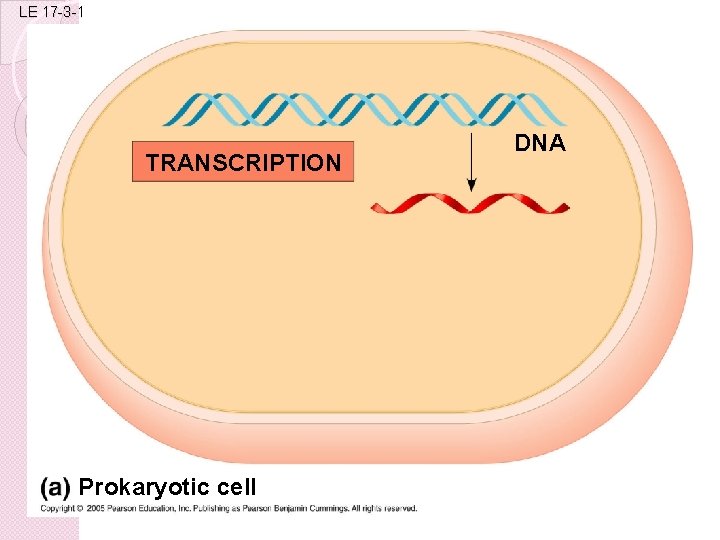
LE 17 -3 -1 TRANSCRIPTION Prokaryotic cell DNA
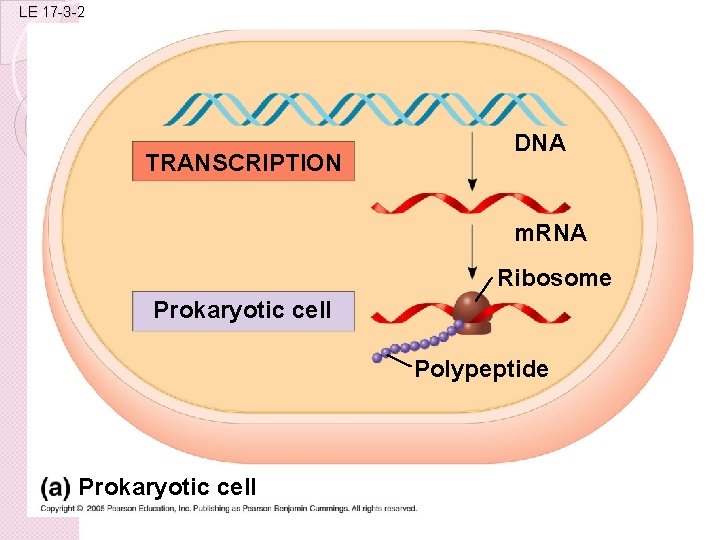
LE 17 -3 -2 TRANSCRIPTION DNA m. RNA Ribosome Prokaryotic cell Polypeptide Prokaryotic cell
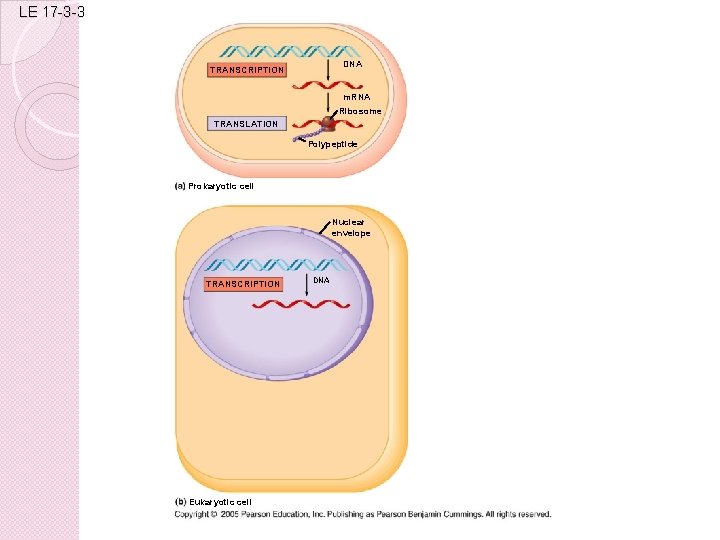
LE 17 -3 -3 DNA TRANSCRIPTION m. RNA Ribosome TRANSLATION Polypeptide Prokaryotic cell Nuclear envelope TRANSCRIPTION Eukaryotic cell DNA
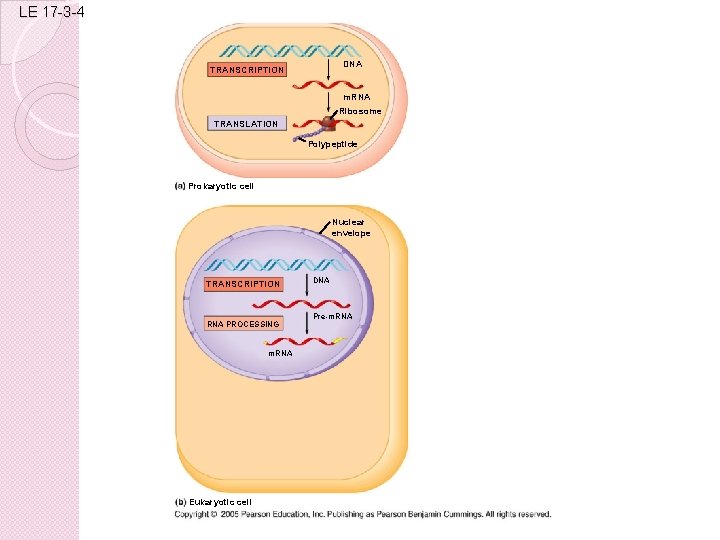
LE 17 -3 -4 DNA TRANSCRIPTION m. RNA Ribosome TRANSLATION Polypeptide Prokaryotic cell Nuclear envelope TRANSCRIPTION RNA PROCESSING m. RNA Eukaryotic cell DNA Pre-m. RNA
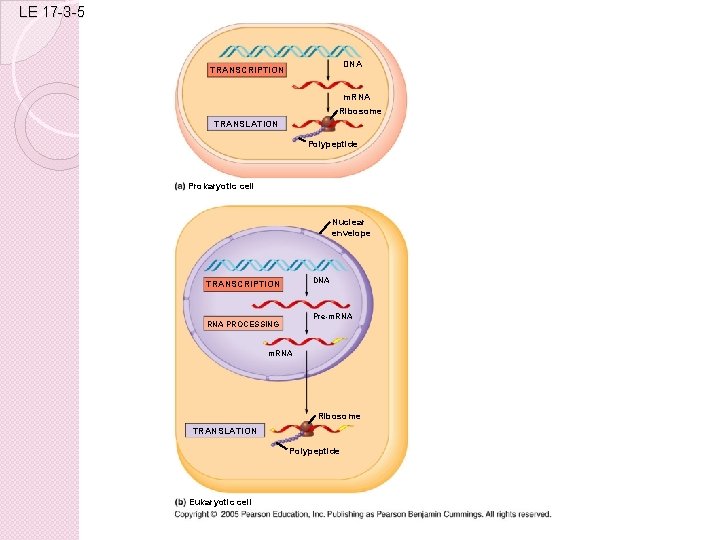
LE 17 -3 -5 DNA TRANSCRIPTION m. RNA Ribosome TRANSLATION Polypeptide Prokaryotic cell Nuclear envelope DNA TRANSCRIPTION Pre-m. RNA PROCESSING m. RNA Ribosome TRANSLATION Polypeptide Eukaryotic cell
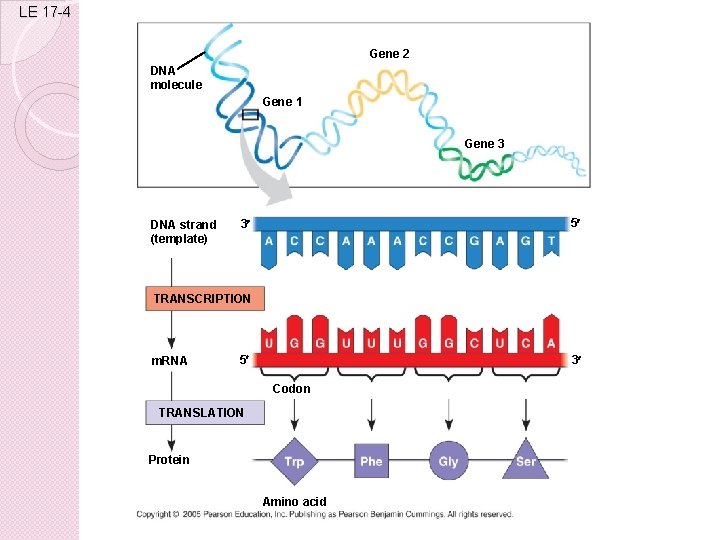
LE 17 -4 Gene 2 DNA molecule Gene 1 Gene 3 DNA strand (template) 5 3 TRANSCRIPTION m. RNA 5 3 Codon TRANSLATION Protein Amino acid
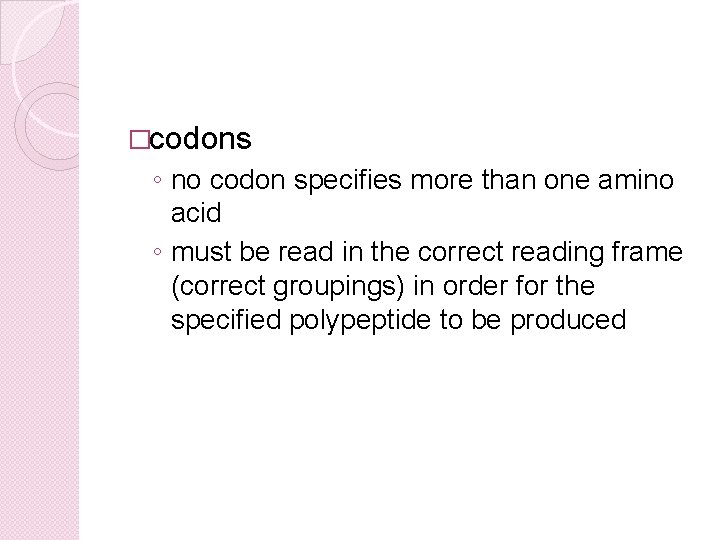
�codons ◦ no codon specifies more than one amino acid ◦ must be read in the correct reading frame (correct groupings) in order for the specified polypeptide to be produced
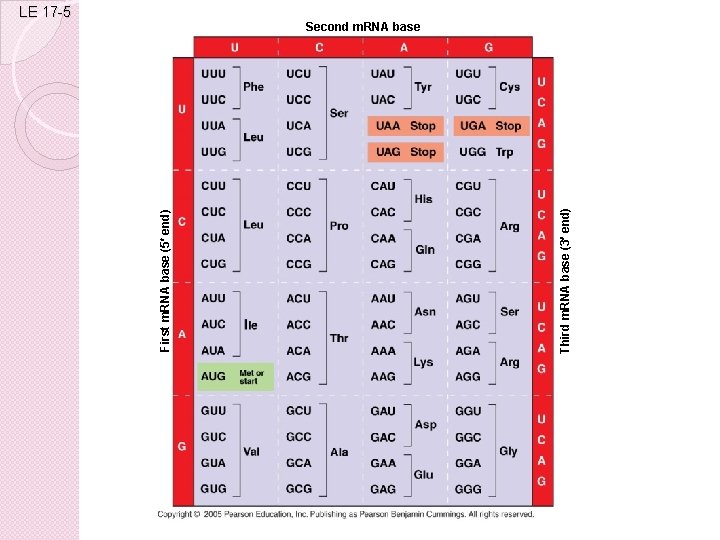
Third m. RNA base (3 end) First m. RNA base (5 end) LE 17 -5 Second m. RNA base
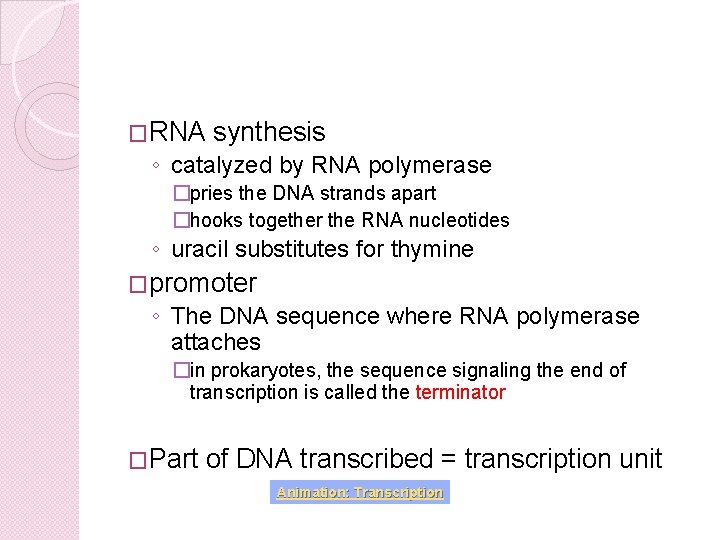
�RNA synthesis ◦ catalyzed by RNA polymerase �pries the DNA strands apart �hooks together the RNA nucleotides ◦ uracil substitutes for thymine �promoter ◦ The DNA sequence where RNA polymerase attaches �in prokaryotes, the sequence signaling the end of transcription is called the terminator �Part of DNA transcribed = transcription unit Animation: Transcription
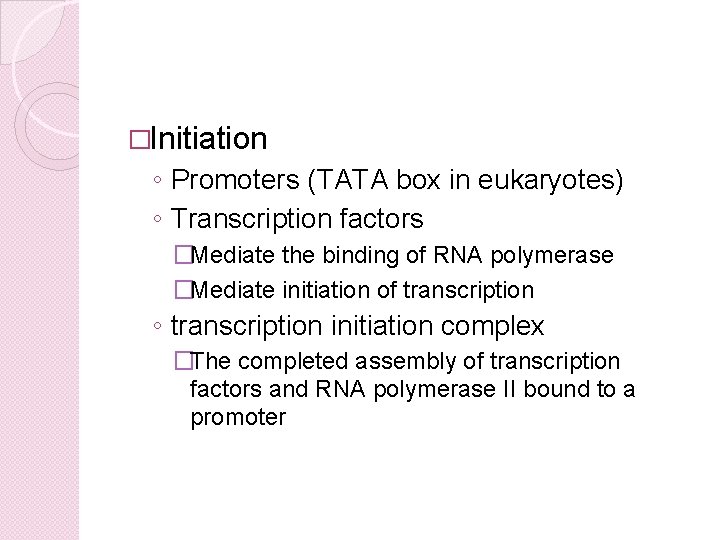
�Initiation ◦ Promoters (TATA box in eukaryotes) ◦ Transcription factors �Mediate the binding of RNA polymerase �Mediate initiation of transcription ◦ transcription initiation complex �The completed assembly of transcription factors and RNA polymerase II bound to a promoter
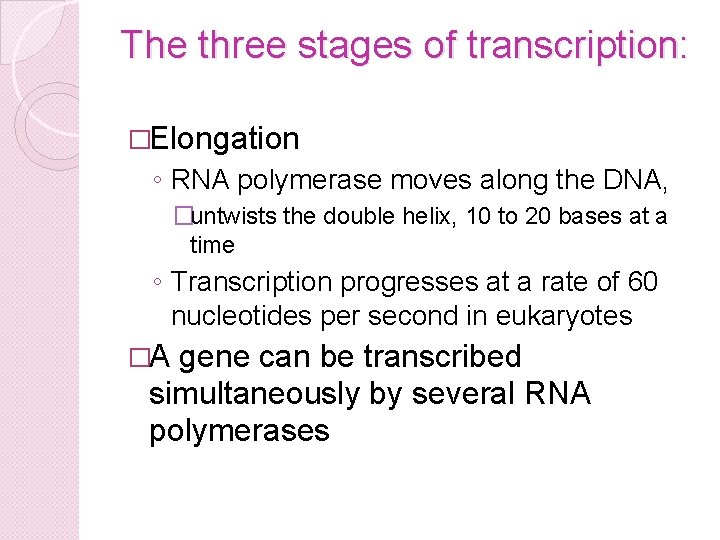
The three stages of transcription: �Elongation ◦ RNA polymerase moves along the DNA, �untwists the double helix, 10 to 20 bases at a time ◦ Transcription progresses at a rate of 60 nucleotides per second in eukaryotes �A gene can be transcribed simultaneously by several RNA polymerases
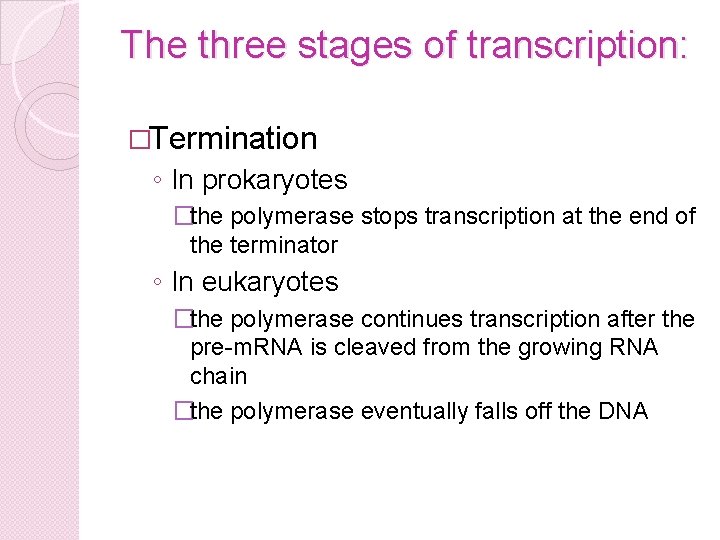
The three stages of transcription: �Termination ◦ In prokaryotes �the polymerase stops transcription at the end of the terminator ◦ In eukaryotes �the polymerase continues transcription after the pre-m. RNA is cleaved from the growing RNA chain �the polymerase eventually falls off the DNA
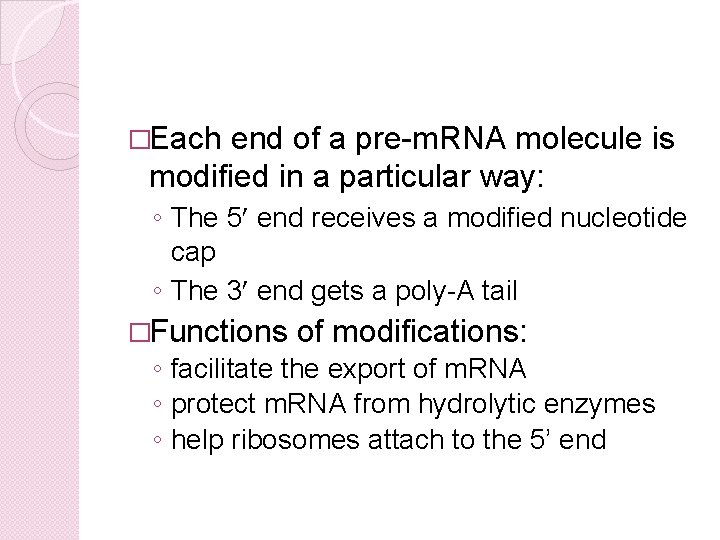
�Each end of a pre-m. RNA molecule is modified in a particular way: ◦ The 5 end receives a modified nucleotide cap ◦ The 3 end gets a poly-A tail �Functions of modifications: ◦ facilitate the export of m. RNA ◦ protect m. RNA from hydrolytic enzymes ◦ help ribosomes attach to the 5’ end
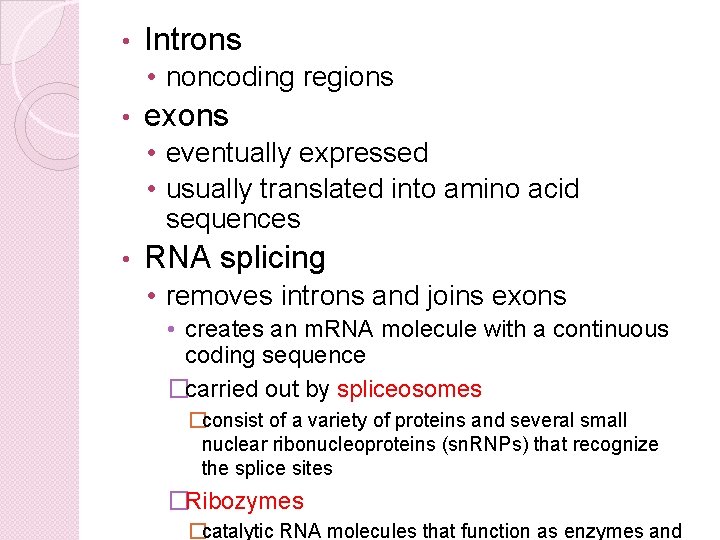
• Introns • noncoding regions • exons • eventually expressed • usually translated into amino acid sequences • RNA splicing • removes introns and joins exons • creates an m. RNA molecule with a continuous coding sequence �carried out by spliceosomes �consist of a variety of proteins and several small nuclear ribonucleoproteins (sn. RNPs) that recognize the splice sites �Ribozymes �catalytic RNA molecules that function as enzymes and
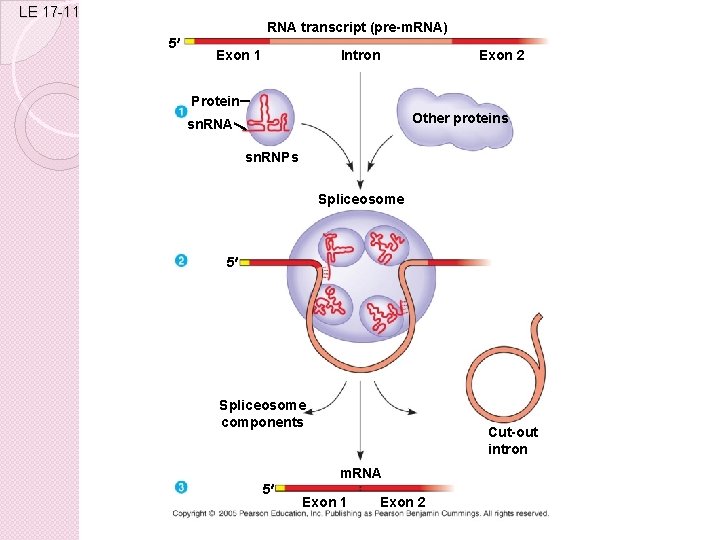
LE 17 -11 RNA transcript (pre-m. RNA) 5 Exon 1 Intron Exon 2 Protein Other proteins sn. RNA sn. RNPs Spliceosome 5 Spliceosome components Cut-out intron m. RNA 5 Exon 1 Exon 2
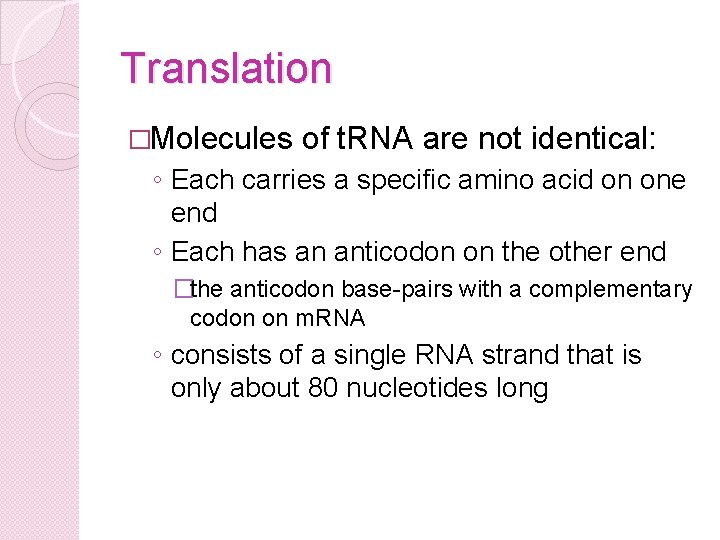
Translation �Molecules of t. RNA are not identical: ◦ Each carries a specific amino acid on one end ◦ Each has an anticodon on the other end �the anticodon base-pairs with a complementary codon on m. RNA ◦ consists of a single RNA strand that is only about 80 nucleotides long
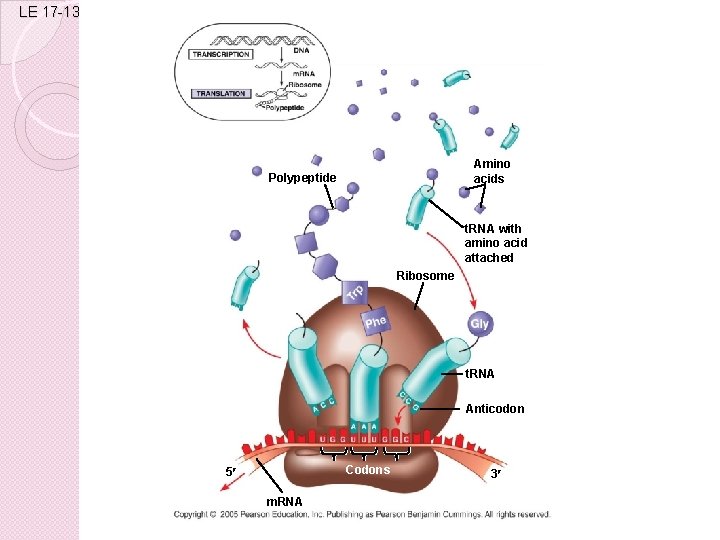
LE 17 -13 Amino acids Polypeptide t. RNA with amino acid attached Ribosome t. RNA Anticodon Codons 5 m. RNA 3
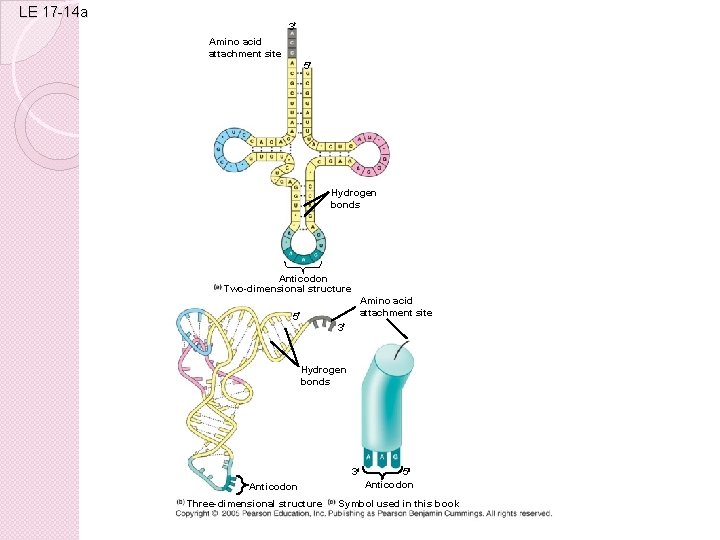
LE 17 -14 a 3 Amino acid attachment site 5 Hydrogen bonds Anticodon Two-dimensional structure Amino acid attachment site 5 3 Hydrogen bonds 3 Anticodon Three-dimensional structure 5 Anticodon Symbol used in this book
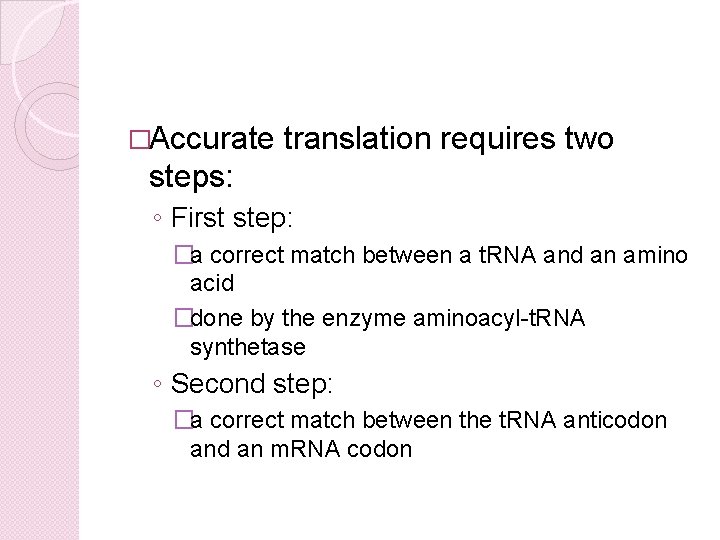
�Accurate translation requires two steps: ◦ First step: �a correct match between a t. RNA and an amino acid �done by the enzyme aminoacyl-t. RNA synthetase ◦ Second step: �a correct match between the t. RNA anticodon and an m. RNA codon
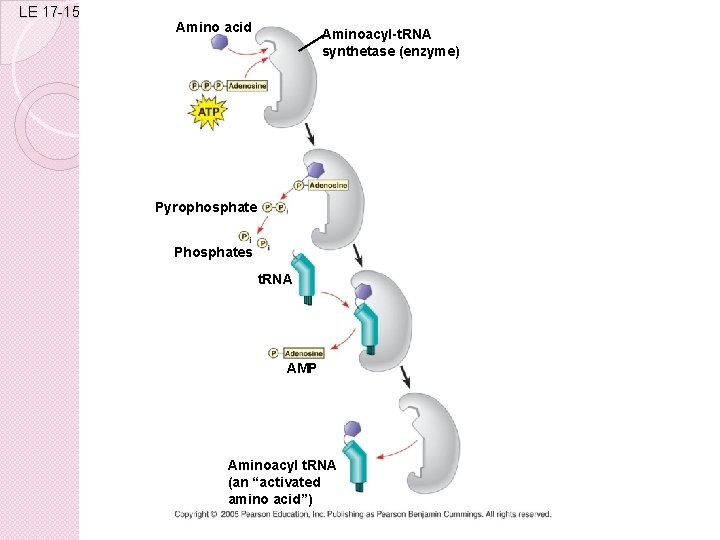
LE 17 -15 Amino acid Aminoacyl-t. RNA synthetase (enzyme) Pyrophosphate Phosphates t. RNA AMP Aminoacyl t. RNA (an “activated amino acid”)
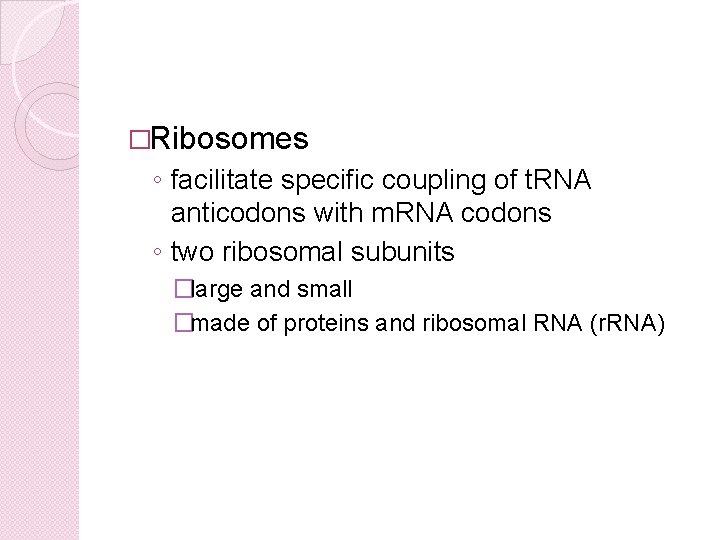
�Ribosomes ◦ facilitate specific coupling of t. RNA anticodons with m. RNA codons ◦ two ribosomal subunits �large and small �made of proteins and ribosomal RNA (r. RNA)
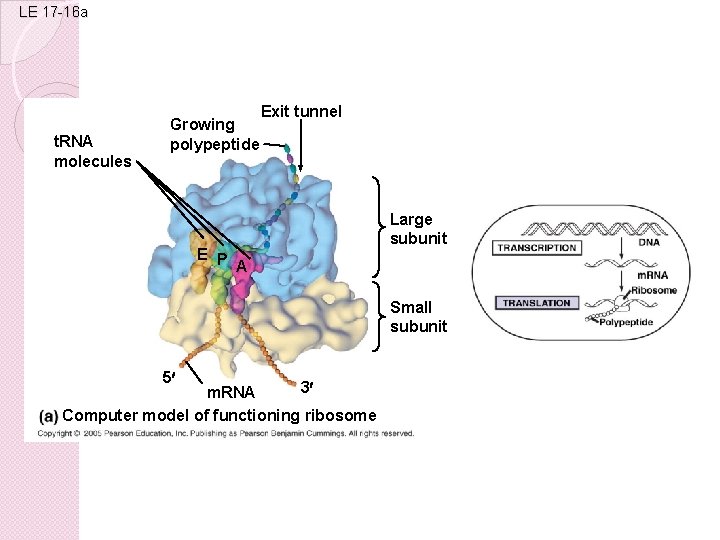
LE 17 -16 a t. RNA molecules Growing polypeptide Exit tunnel E P A Large subunit Small subunit 5 3 m. RNA Computer model of functioning ribosome
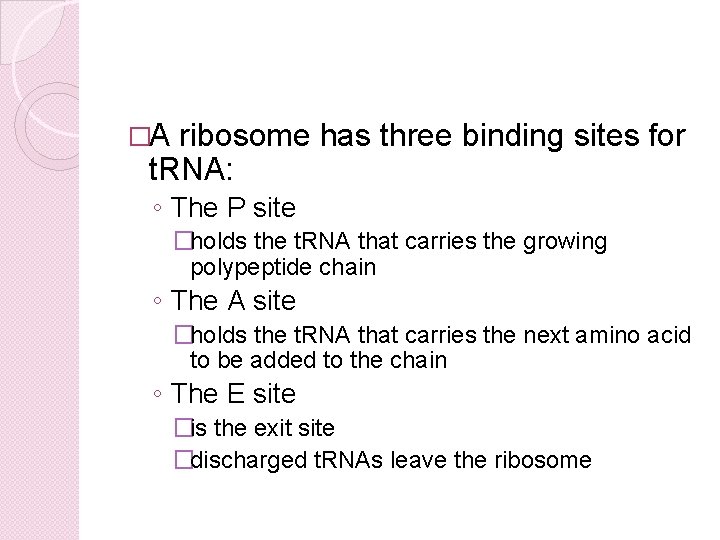
�A ribosome has three binding sites for t. RNA: ◦ The P site �holds the t. RNA that carries the growing polypeptide chain ◦ The A site �holds the t. RNA that carries the next amino acid to be added to the chain ◦ The E site �is the exit site �discharged t. RNAs leave the ribosome
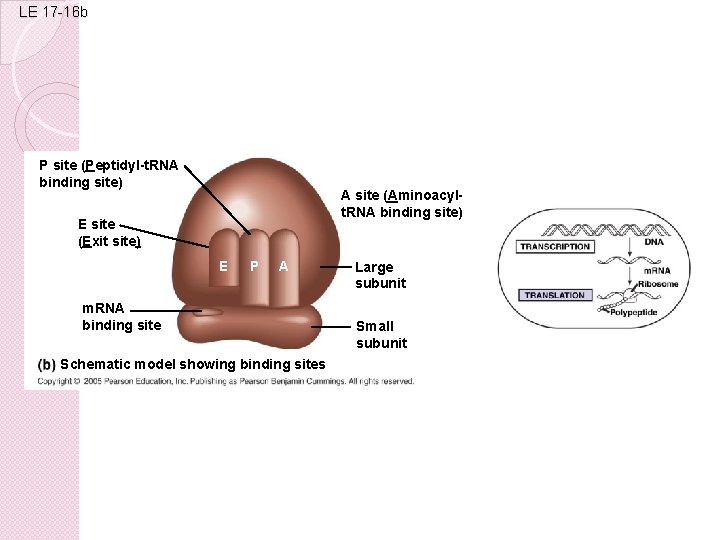
LE 17 -16 b P site (Peptidyl-t. RNA binding site) A site (Aminoacylt. RNA binding site) E site (Exit site) E P A m. RNA binding site Schematic model showing binding sites Large subunit Small subunit
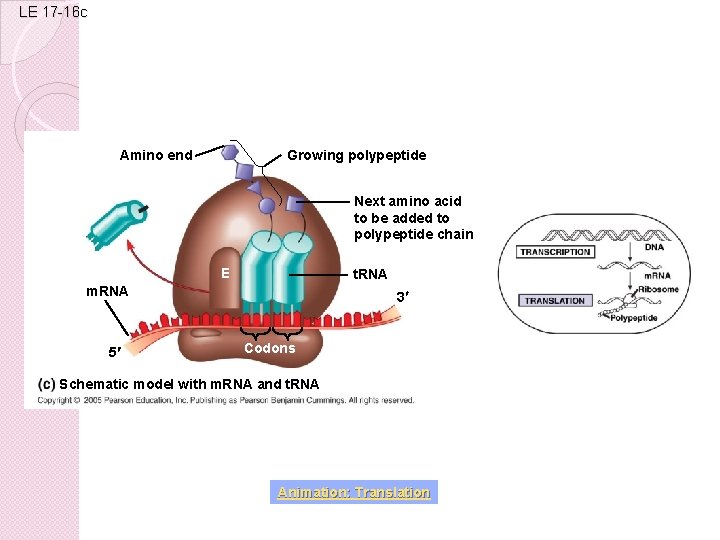
LE 17 -16 c Amino end Growing polypeptide Next amino acid to be added to polypeptide chain E t. RNA m. RNA 5 3 Codons Schematic model with m. RNA and t. RNA Animation: Translation
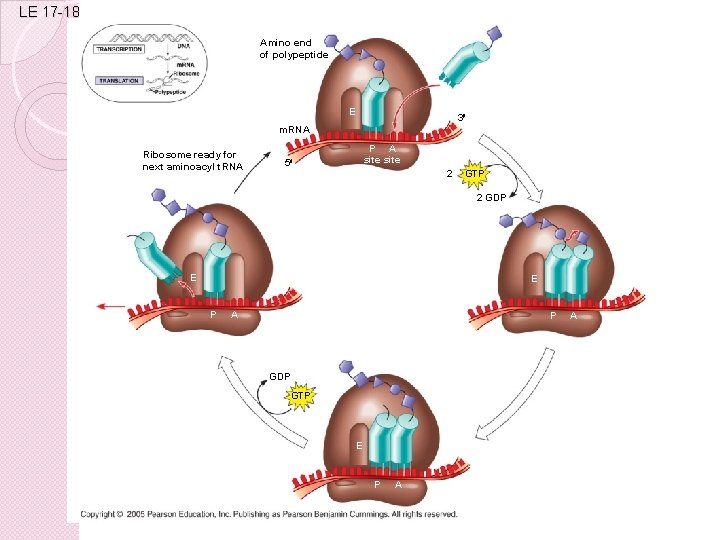
LE 17 -18 Amino end of polypeptide E 3 m. RNA Ribosome ready for next aminoacyl t. RNA P A site 5 2 GTP 2 GDP E E P A P GDP GTP E P A A
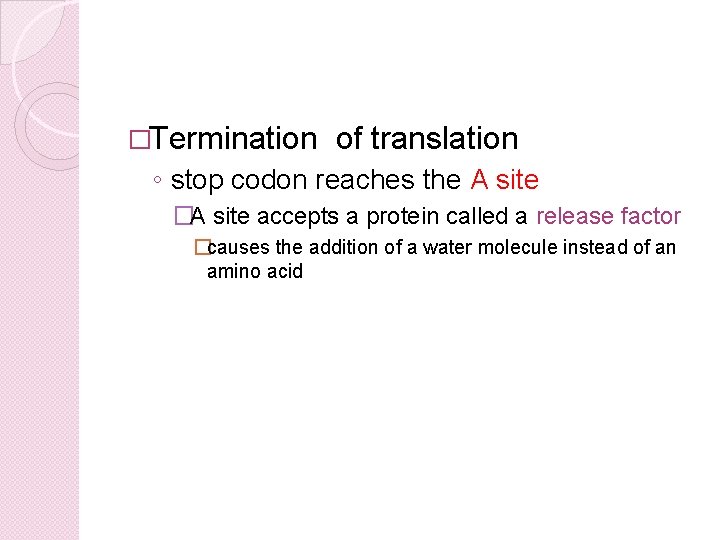
�Termination of translation ◦ stop codon reaches the A site �A site accepts a protein called a release factor �causes the addition of a water molecule instead of an amino acid
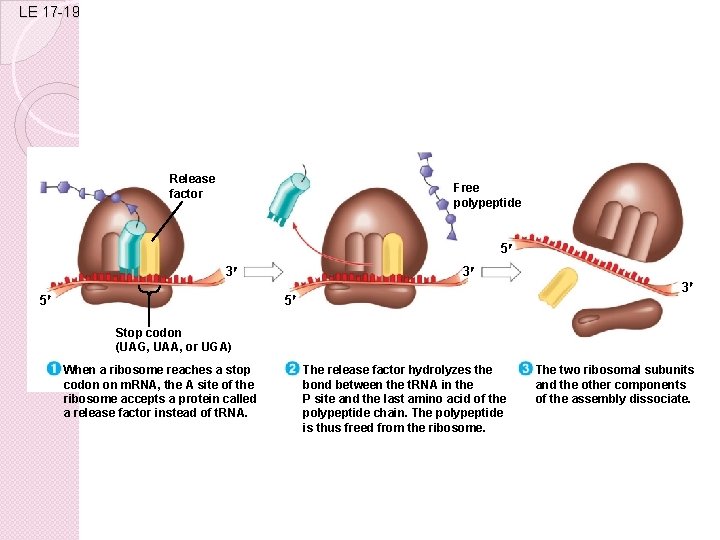
LE 17 -19 Release factor Free polypeptide 5 3 5 3 3 5 Stop codon (UAG, UAA, or UGA) When a ribosome reaches a stop codon on m. RNA, the A site of the ribosome accepts a protein called a release factor instead of t. RNA. The release factor hydrolyzes the bond between the t. RNA in the P site and the last amino acid of the polypeptide chain. The polypeptide is thus freed from the ribosome. The two ribosomal subunits and the other components of the assembly dissociate.
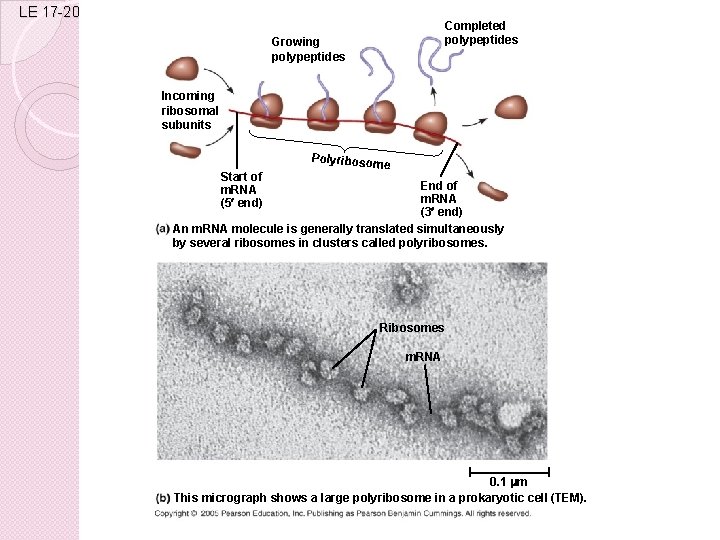
LE 17 -20 Completed polypeptides Growing polypeptides Incoming ribosomal subunits Polyriboso Start of m. RNA (5 end) me End of m. RNA (3 end) An m. RNA molecule is generally translated simultaneously by several ribosomes in clusters called polyribosomes. Ribosomes m. RNA 0. 1 mm This micrograph shows a large polyribosome in a prokaryotic cell (TEM).
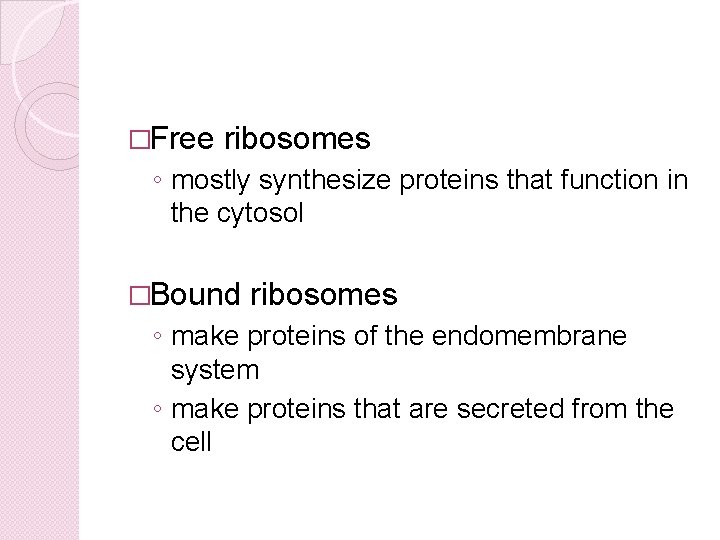
�Free ribosomes ◦ mostly synthesize proteins that function in the cytosol �Bound ribosomes ◦ make proteins of the endomembrane system ◦ make proteins that are secreted from the cell
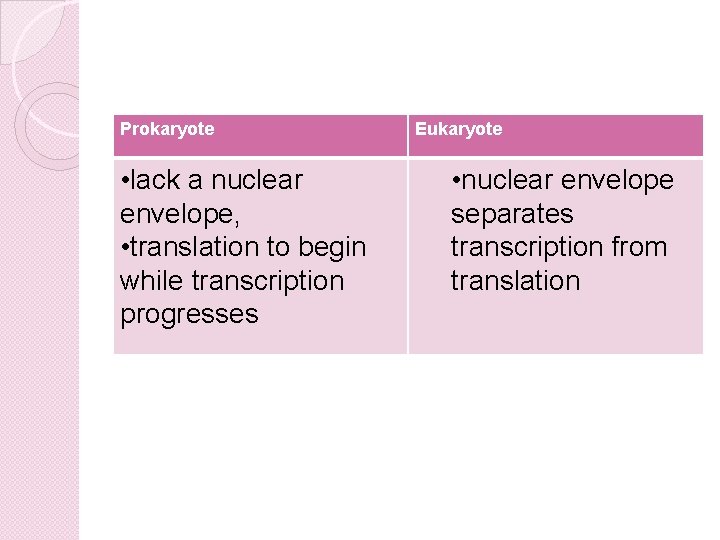
Prokaryote • lack a nuclear envelope, • translation to begin while transcription progresses Eukaryote • nuclear envelope separates transcription from translation
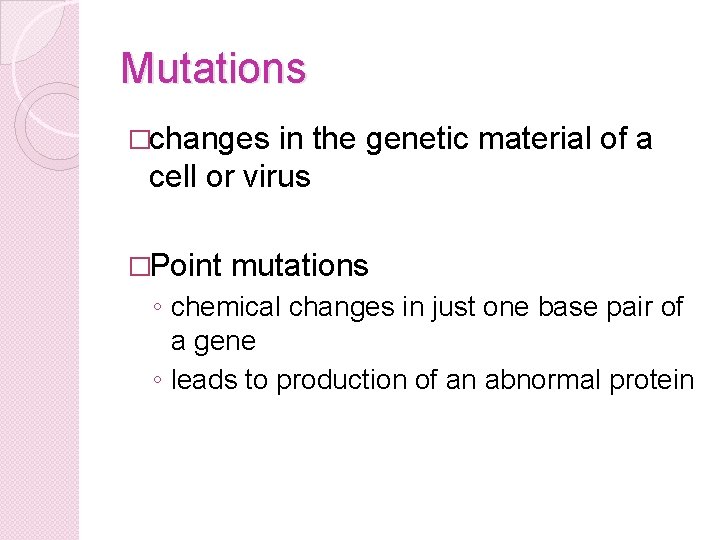
Mutations �changes in the genetic material of a cell or virus �Point mutations ◦ chemical changes in just one base pair of a gene ◦ leads to production of an abnormal protein
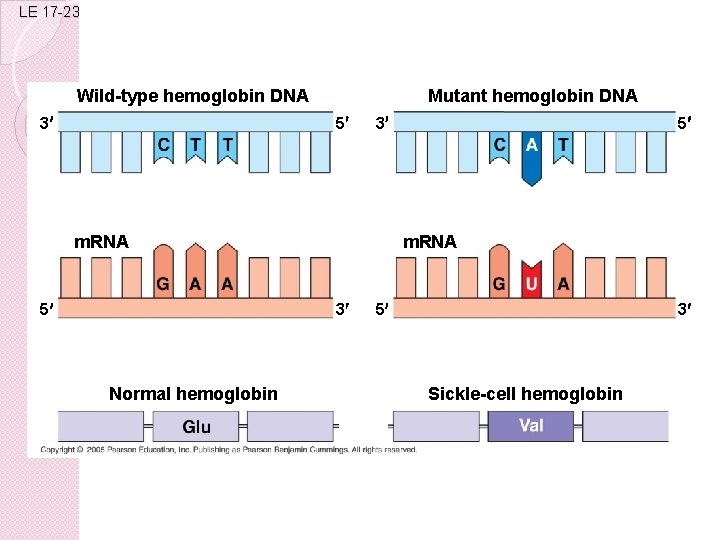
LE 17 -23 Wild-type hemoglobin DNA 3 Mutant hemoglobin DNA 5 3 m. RNA 5 m. RNA 3 Normal hemoglobin 5 5 3 Sickle-cell hemoglobin
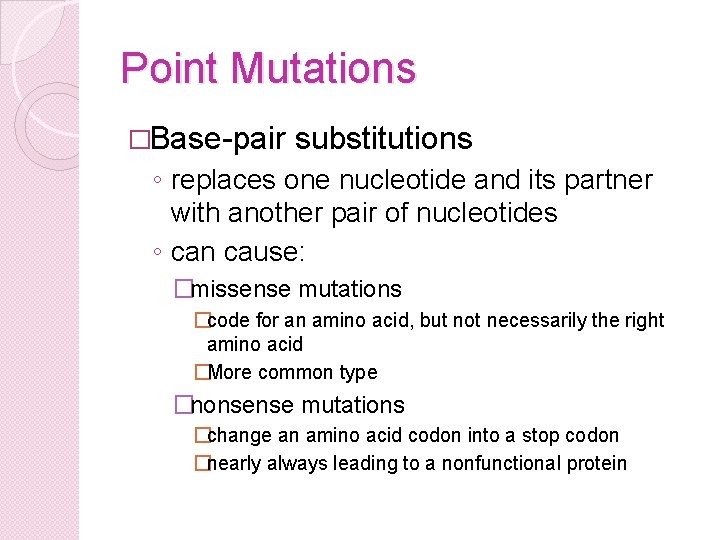
Point Mutations �Base-pair substitutions ◦ replaces one nucleotide and its partner with another pair of nucleotides ◦ can cause: �missense mutations �code for an amino acid, but not necessarily the right amino acid �More common type �nonsense mutations �change an amino acid codon into a stop codon �nearly always leading to a nonfunctional protein
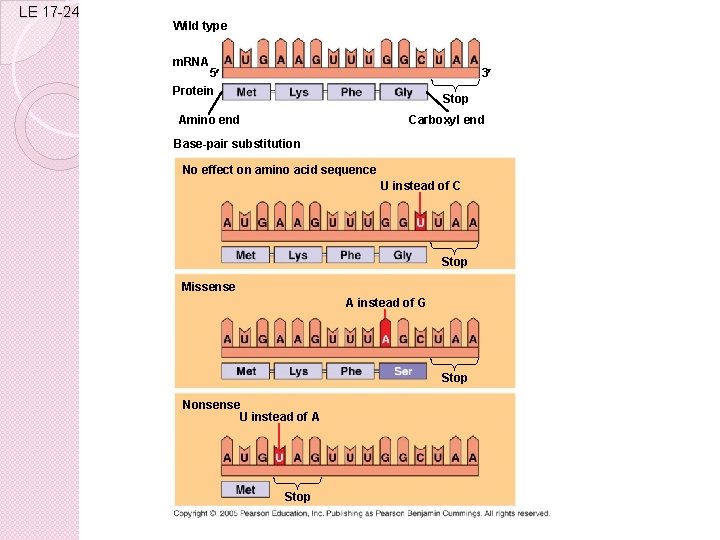
LE 17 -24 Wild type m. RNA 5 Protein 3 Stop Amino end Carboxyl end Base-pair substitution No effect on amino acid sequence U instead of C Stop Missense A instead of G Stop Nonsense U instead of A Stop
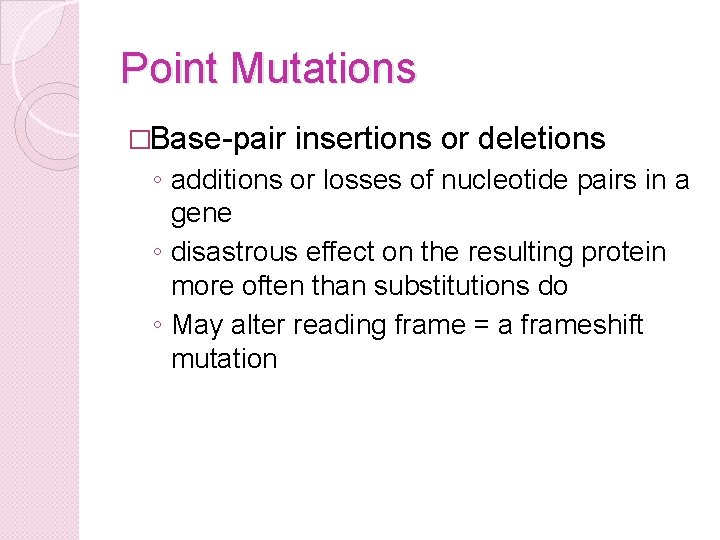
Point Mutations �Base-pair insertions or deletions ◦ additions or losses of nucleotide pairs in a gene ◦ disastrous effect on the resulting protein more often than substitutions do ◦ May alter reading frame = a frameshift mutation
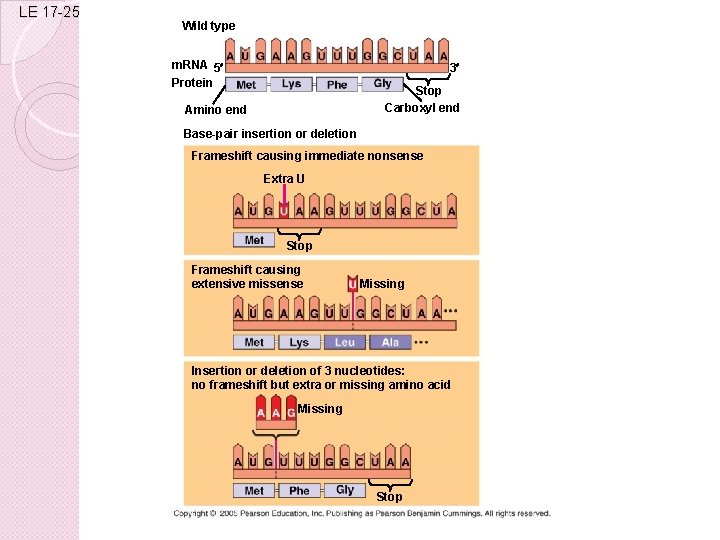
LE 17 -25 Wild type m. RNA 5 Protein 3 Stop Carboxyl end Amino end Base-pair insertion or deletion Frameshift causing immediate nonsense Extra U Stop Frameshift causing extensive missense Missing Insertion or deletion of 3 nucleotides: no frameshift but extra or missing amino acid Missing Stop
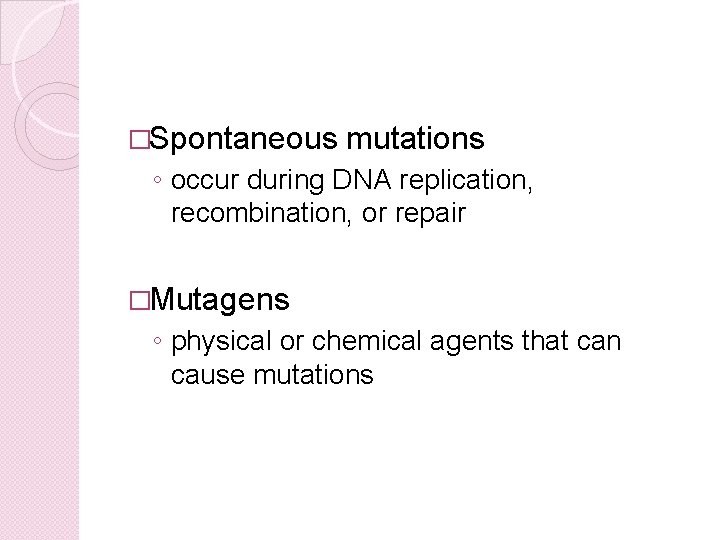
�Spontaneous mutations ◦ occur during DNA replication, recombination, or repair �Mutagens ◦ physical or chemical agents that can cause mutations
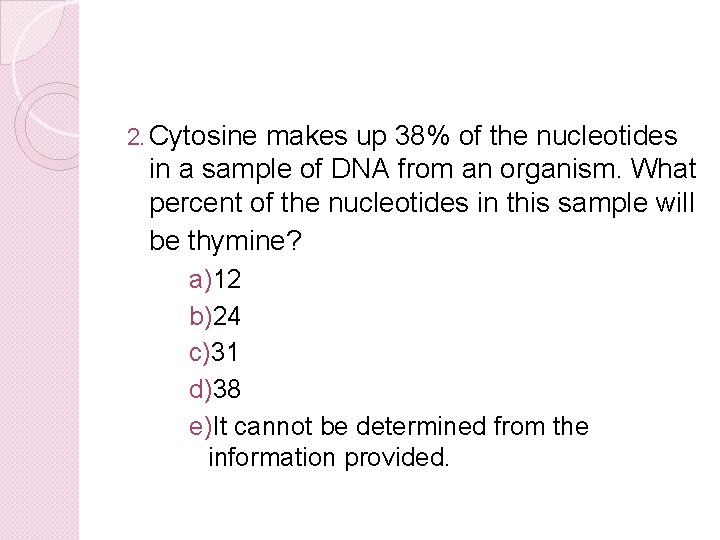
2. Cytosine makes up 38% of the nucleotides in a sample of DNA from an organism. What percent of the nucleotides in this sample will be thymine? a)12 b)24 c)31 d)38 e)It cannot be determined from the information provided.
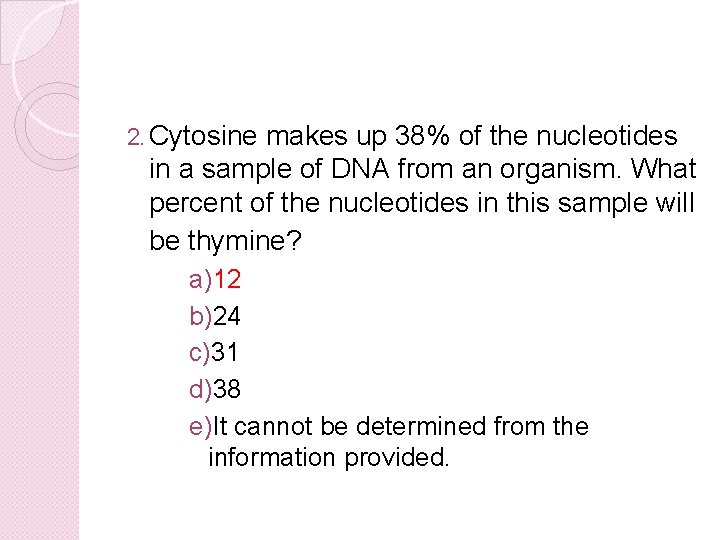
2. Cytosine makes up 38% of the nucleotides in a sample of DNA from an organism. What percent of the nucleotides in this sample will be thymine? a)12 b)24 c)31 d)38 e)It cannot be determined from the information provided.
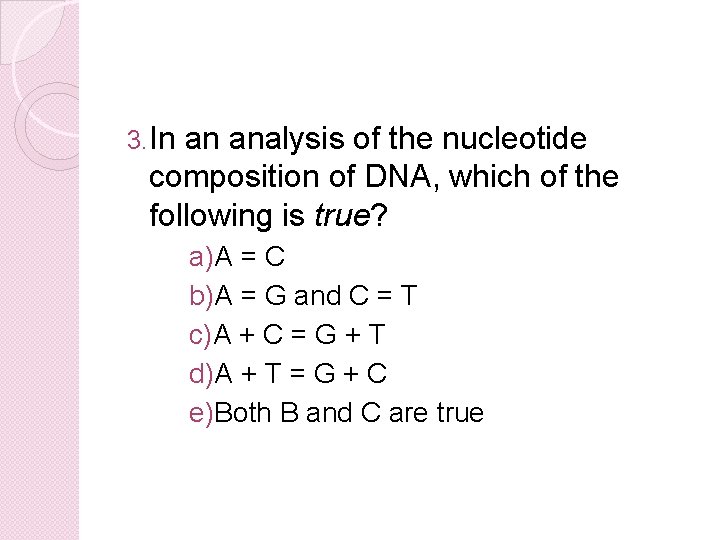
3. In an analysis of the nucleotide composition of DNA, which of the following is true? a)A = C b)A = G and C = T c)A + C = G + T d)A + T = G + C e)Both B and C are true
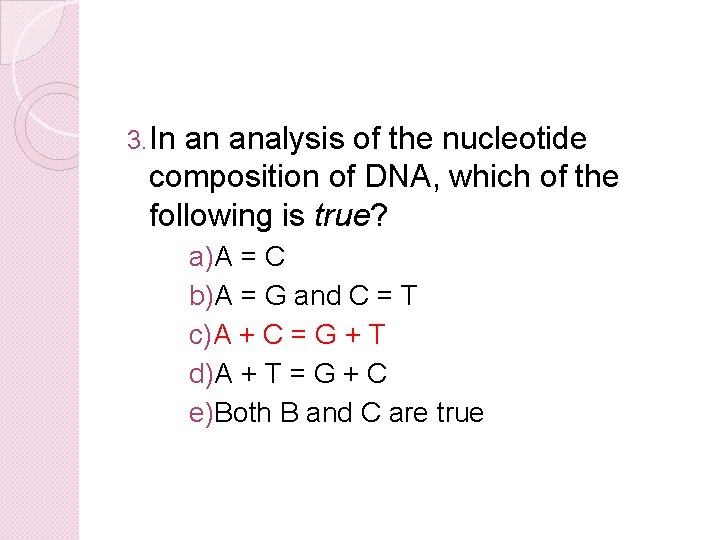
3. In an analysis of the nucleotide composition of DNA, which of the following is true? a)A = C b)A = G and C = T c)A + C = G + T d)A + T = G + C e)Both B and C are true
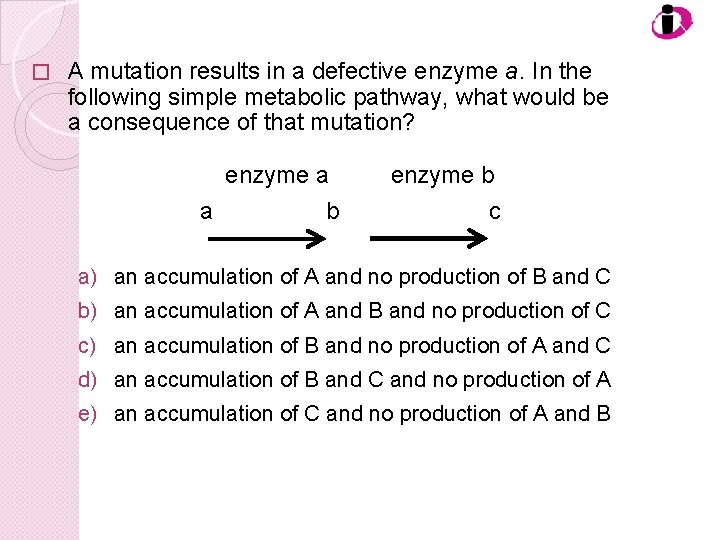
� A mutation results in a defective enzyme a. In the following simple metabolic pathway, what would be a consequence of that mutation? enzyme a a b enzyme b c a) an accumulation of A and no production of B and C b) an accumulation of A and B and no production of C c) an accumulation of B and no production of A and C d) an accumulation of B and C and no production of A e) an accumulation of C and no production of A and B
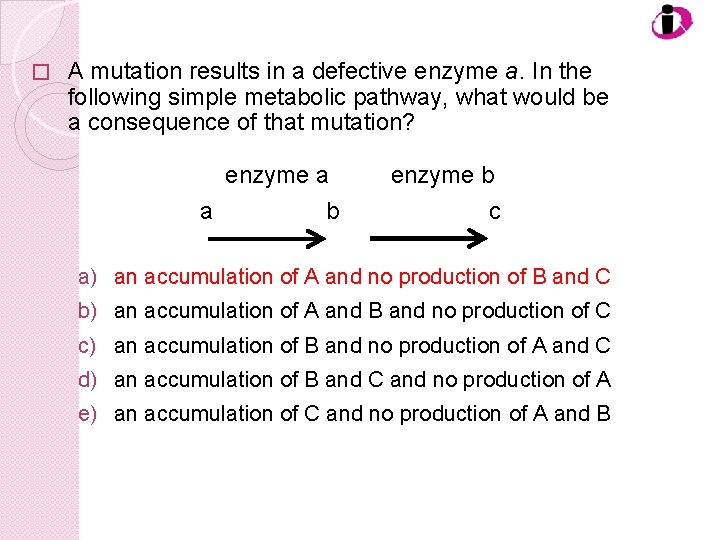
� A mutation results in a defective enzyme a. In the following simple metabolic pathway, what would be a consequence of that mutation? enzyme a a b enzyme b c a) an accumulation of A and no production of B and C b) an accumulation of A and B and no production of C c) an accumulation of B and no production of A and C d) an accumulation of B and C and no production of A e) an accumulation of C and no production of A and B
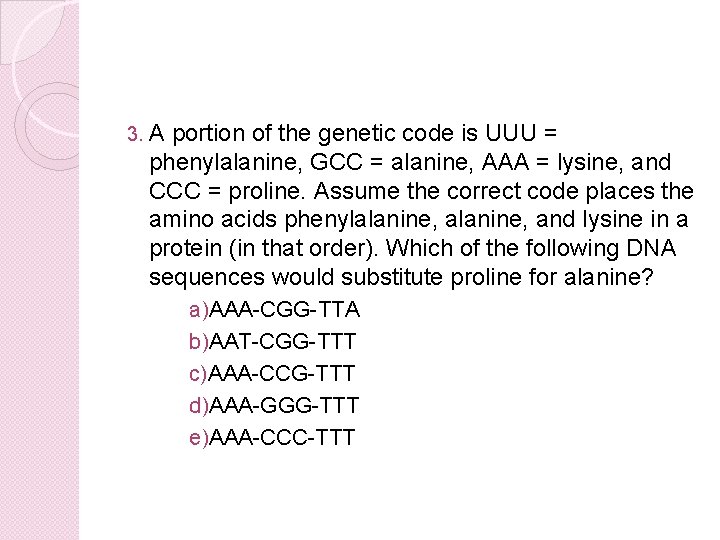
3. A portion of the genetic code is UUU = phenylalanine, GCC = alanine, AAA = lysine, and CCC = proline. Assume the correct code places the amino acids phenylalanine, and lysine in a protein (in that order). Which of the following DNA sequences would substitute proline for alanine? a)AAA-CGG-TTA b)AAT-CGG-TTT c)AAA-CCG-TTT d)AAA-GGG-TTT e)AAA-CCC-TTT
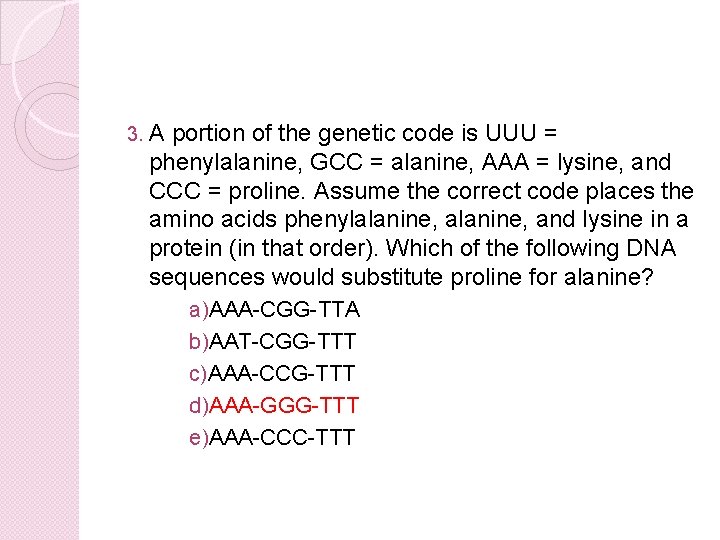
3. A portion of the genetic code is UUU = phenylalanine, GCC = alanine, AAA = lysine, and CCC = proline. Assume the correct code places the amino acids phenylalanine, and lysine in a protein (in that order). Which of the following DNA sequences would substitute proline for alanine? a)AAA-CGG-TTA b)AAT-CGG-TTT c)AAA-CCG-TTT d)AAA-GGG-TTT e)AAA-CCC-TTT
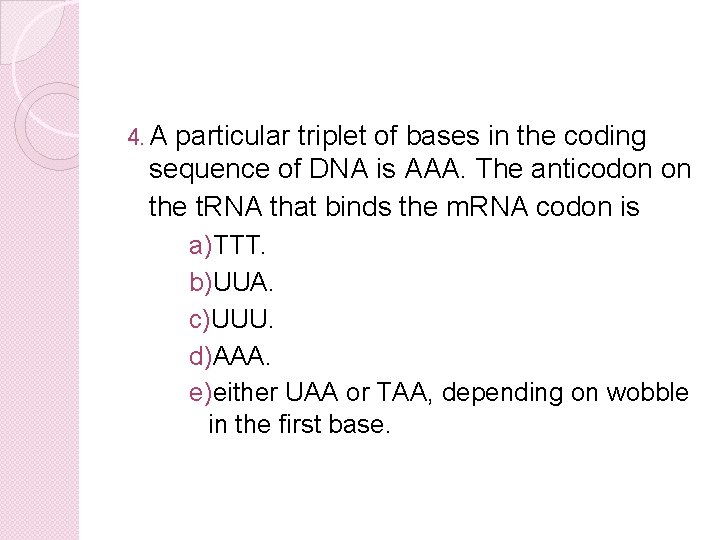
4. A particular triplet of bases in the coding sequence of DNA is AAA. The anticodon on the t. RNA that binds the m. RNA codon is a)TTT. b)UUA. c)UUU. d)AAA. e)either UAA or TAA, depending on wobble in the first base.
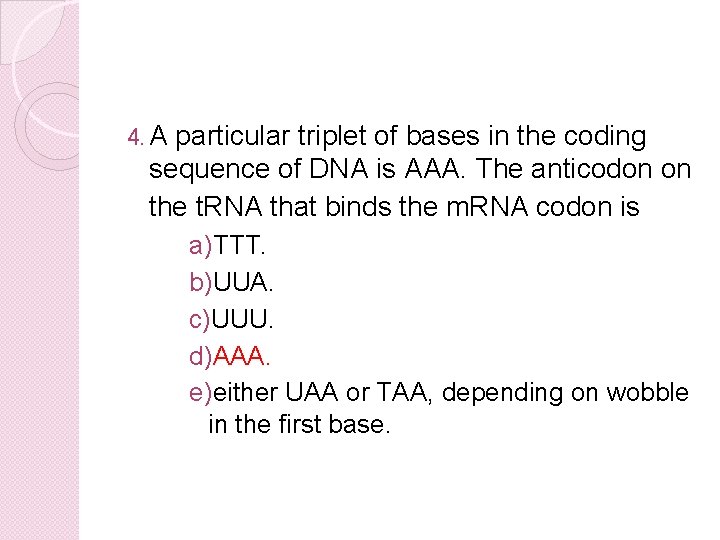
4. A particular triplet of bases in the coding sequence of DNA is AAA. The anticodon on the t. RNA that binds the m. RNA codon is a)TTT. b)UUA. c)UUU. d)AAA. e)either UAA or TAA, depending on wobble in the first base.
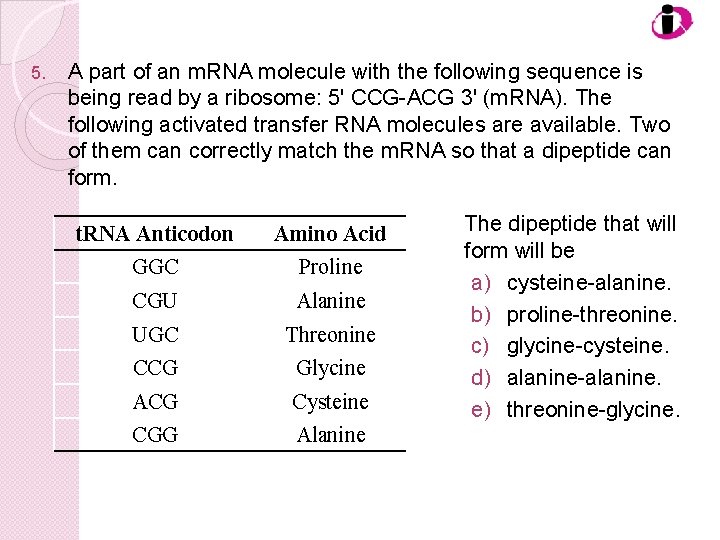
5. A part of an m. RNA molecule with the following sequence is being read by a ribosome: 5' CCG-ACG 3' (m. RNA). The following activated transfer RNA molecules are available. Two of them can correctly match the m. RNA so that a dipeptide can form. t. RNA Anticodon Amino Acid GGC Proline CGU Alanine UGC Threonine CCG Glycine ACG Cysteine CGG Alanine The dipeptide that will form will be a) cysteine-alanine. b) proline-threonine. c) glycine-cysteine. d) alanine-alanine. e) threonine-glycine.
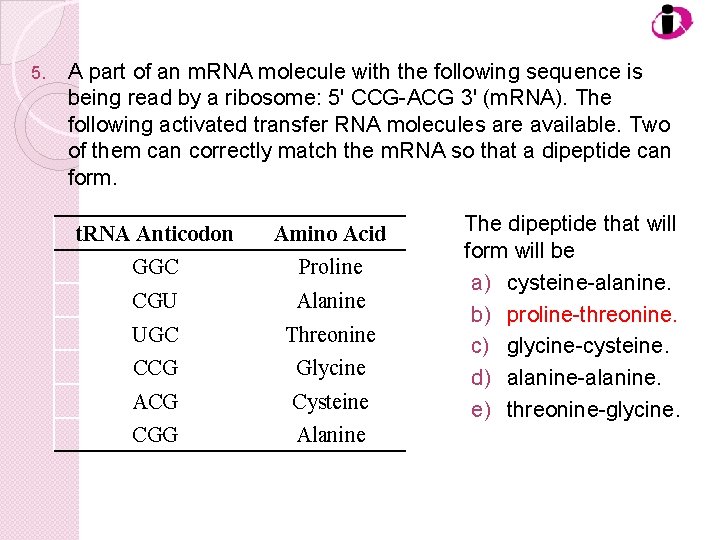
5. A part of an m. RNA molecule with the following sequence is being read by a ribosome: 5' CCG-ACG 3' (m. RNA). The following activated transfer RNA molecules are available. Two of them can correctly match the m. RNA so that a dipeptide can form. t. RNA Anticodon Amino Acid GGC Proline CGU Alanine UGC Threonine CCG Glycine ACG Cysteine CGG Alanine The dipeptide that will form will be a) cysteine-alanine. b) proline-threonine. c) glycine-cysteine. d) alanine-alanine. e) threonine-glycine.
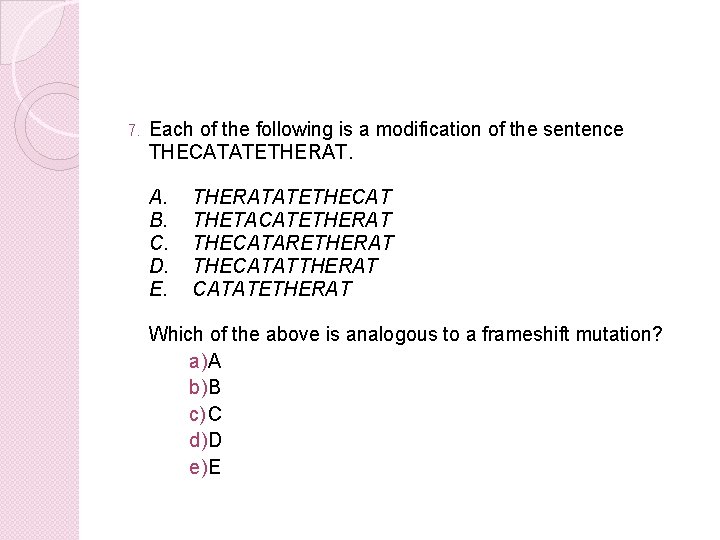
7. Each of the following is a modification of the sentence THECATATETHERAT. A. B. C. D. E. THERATATETHECAT THETACATETHERAT THECATARETHERAT THECATATTHERAT CATATETHERAT Which of the above is analogous to a frameshift mutation? a)A b)B c) C d)D e)E
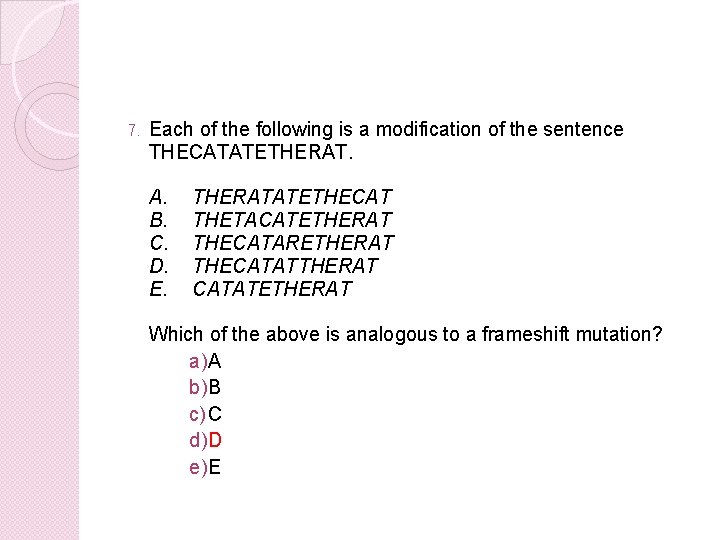
7. Each of the following is a modification of the sentence THECATATETHERAT. A. B. C. D. E. THERATATETHECAT THETACATETHERAT THECATARETHERAT THECATATTHERAT CATATETHERAT Which of the above is analogous to a frameshift mutation? a)A b)B c) C d)D e)E
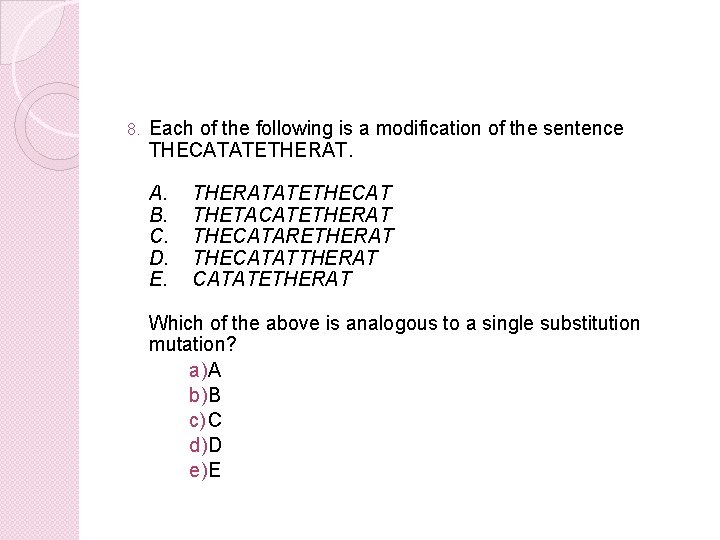
8. Each of the following is a modification of the sentence THECATATETHERAT. A. B. C. D. E. THERATATETHECAT THETACATETHERAT THECATARETHERAT THECATATTHERAT CATATETHERAT Which of the above is analogous to a single substitution mutation? a)A b)B c) C d)D e)E
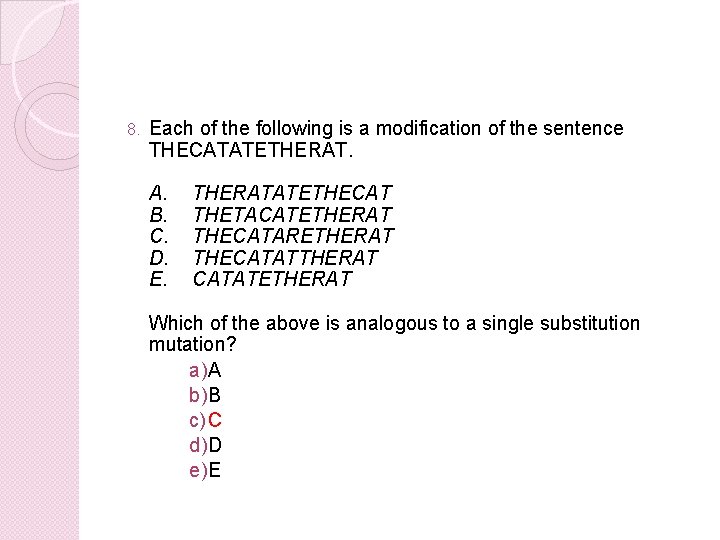
8. Each of the following is a modification of the sentence THECATATETHERAT. A. B. C. D. E. THERATATETHECAT THETACATETHERAT THECATARETHERAT THECATATTHERAT CATATETHERAT Which of the above is analogous to a single substitution mutation? a)A b)B c) C d)D e)E
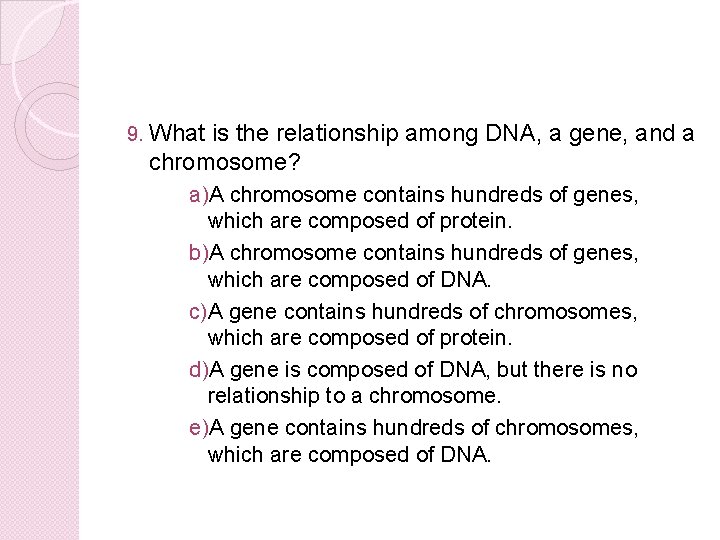
9. What is the relationship among DNA, a gene, and a chromosome? a)A chromosome contains hundreds of genes, which are composed of protein. b)A chromosome contains hundreds of genes, which are composed of DNA. c)A gene contains hundreds of chromosomes, which are composed of protein. d)A gene is composed of DNA, but there is no relationship to a chromosome. e)A gene contains hundreds of chromosomes, which are composed of DNA.
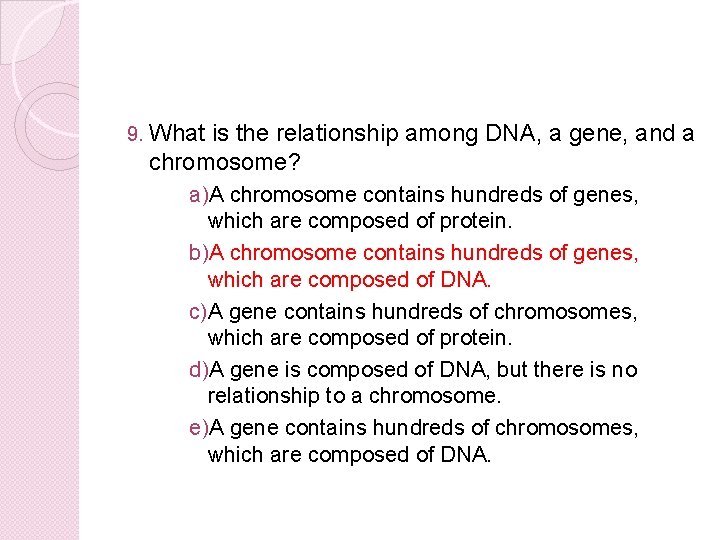
9. What is the relationship among DNA, a gene, and a chromosome? a)A chromosome contains hundreds of genes, which are composed of protein. b)A chromosome contains hundreds of genes, which are composed of DNA. c)A gene contains hundreds of chromosomes, which are composed of protein. d)A gene is composed of DNA, but there is no relationship to a chromosome. e)A gene contains hundreds of chromosomes, which are composed of DNA.
Dna rna protein synthesis homework #2 dna replication
Dna protein synthesis study guide answers
The major key players on the international side are the
The major key players on the international side are the:
Financial accounting standards
The major key players on the international side are the
Rna protein synthesis
Section 12-3 rna and protein synthesis
Rna protein synthesis
Cell analogy restaurant
Transcription and translation
Protein synthesis bbc bitesize
Protein synthesis and mutations
Protein synthesis cookie analogy
Elongation
Rna types
Protein synthesis
Protein synthesis
Protein synthesis and mutations
Protein synthesis story
Protein synthesis and mutations
Protein synthesis animation mcgraw hill
Molecular genetics and biotechnology
Translation protein synthesis
Ribonucleic acid
Ribosome
Protein synthesis ppt
Transcription and translation picture
Protein synthesis inhibitor
Which best summarizes the process of protein synthesis?
Concept map of protein synthesis
Protein synthesis
Protein synthesis scramble
Process of protein synthesis
Dna rna
Teste de ames
Protein synthesis
Protein synthesis
Protein synthesis
Protein synthesis
Catalytic functions
What provides instructions for protein synthesis
Section 12 3 rna and protein synthesis
Channel vs carrier proteins
Protein-protein docking
Bioflix activity dna replication lagging strand synthesis
Dna and rna diagram
Dna-templated synthesis
Dna synthesis at replication fork
Telomerase
Dna to protein steps
Mutations quiz
Chapter 12 section 3 dna rna and protein
Dna to protein steps
Dna to protein steps
Dna rna protein
Dna rna protein
Dna rna protein diagram
Dna-protein interactions
Dna rna protein trait
Function of dna polymerase 3
Coding dna and non coding dna
The principal enzyme involved in dna replication is
Synthesizing information allows a reader to
Major enzymes in dna replication
Major minor groove
Humans share 50 of their dna with bananas
Which organelle breaks down
Muhammad islam founder
Embouchure tips for brass players
Irish american baseball players
Colin took a sample of 80 football players
Rules of snooker
Evolution of music players
Characteristics of sustainable tourism
Key players in cruise industry
The three players in the money supply process include
Why is agility important in tennis
Lorenzo neal wrestling
Team vs working group
Pinpeat ensemble instruments
A basketball is being pushed by two players
Collective noun for basketball players
Nau hockey team
Football players that wrestled
2 types of main idea
Opening knight players
Key players in malaysian industrial relations
Players weebly
Periodic table of soccer players