RF Cavity Resonator and Components ENEA Frascati 3112016
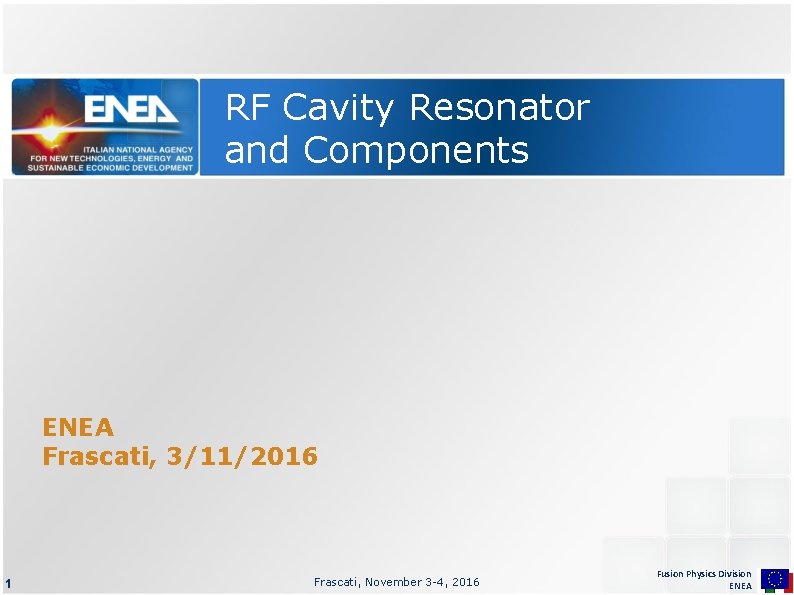
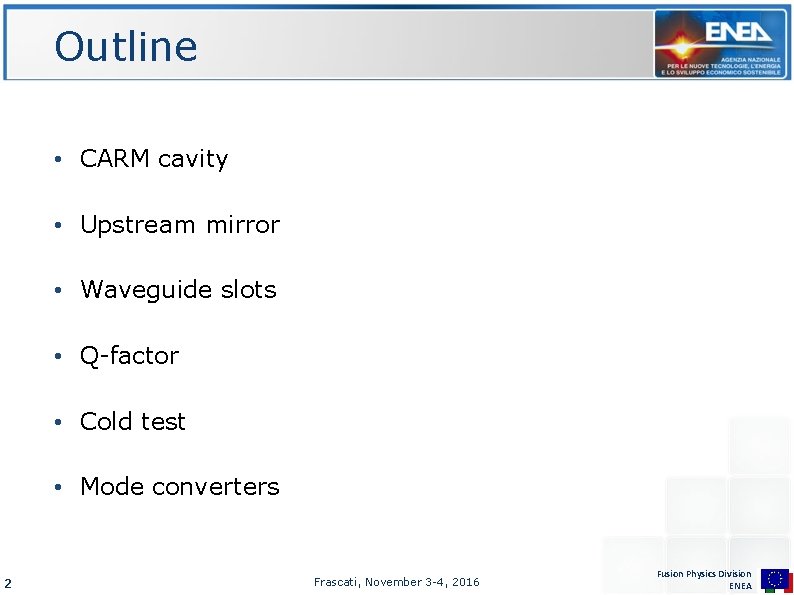
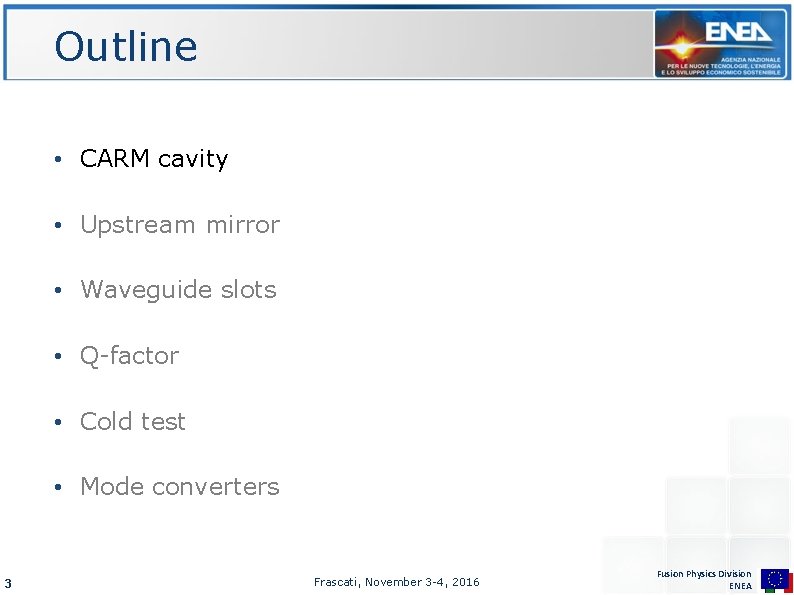
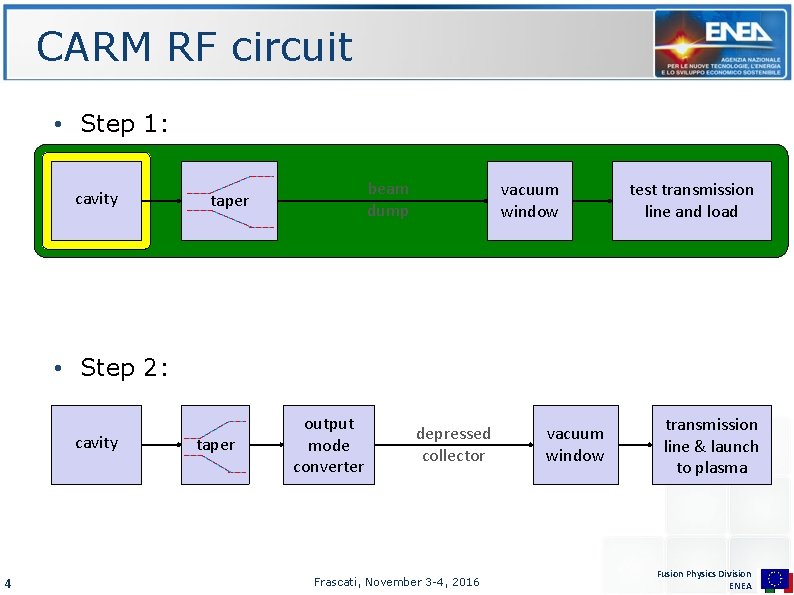
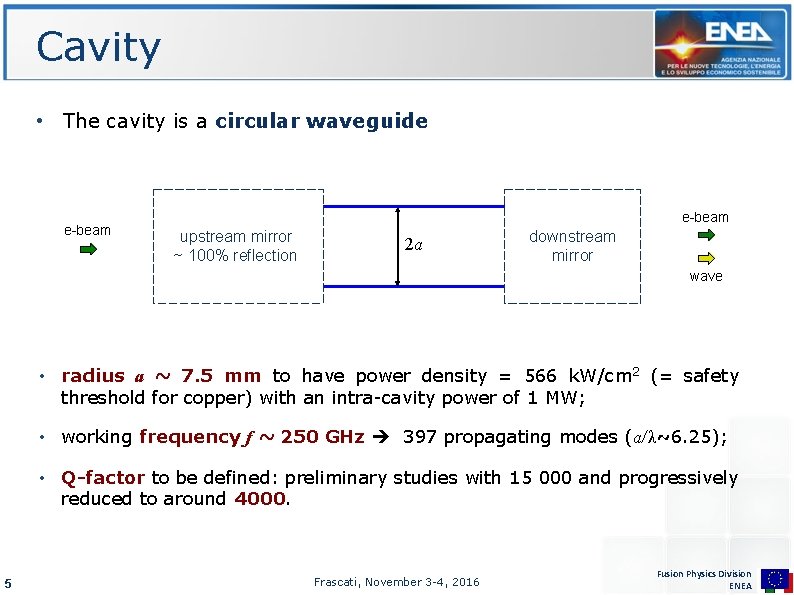
![Cavity options • Quasi-optical resonator [1]: high thermal load on discrete mirrors • Bragg Cavity options • Quasi-optical resonator [1]: high thermal load on discrete mirrors • Bragg](https://slidetodoc.com/presentation_image_h2/9c82b675c66be87ef7f1b4dc394a2855/image-6.jpg)
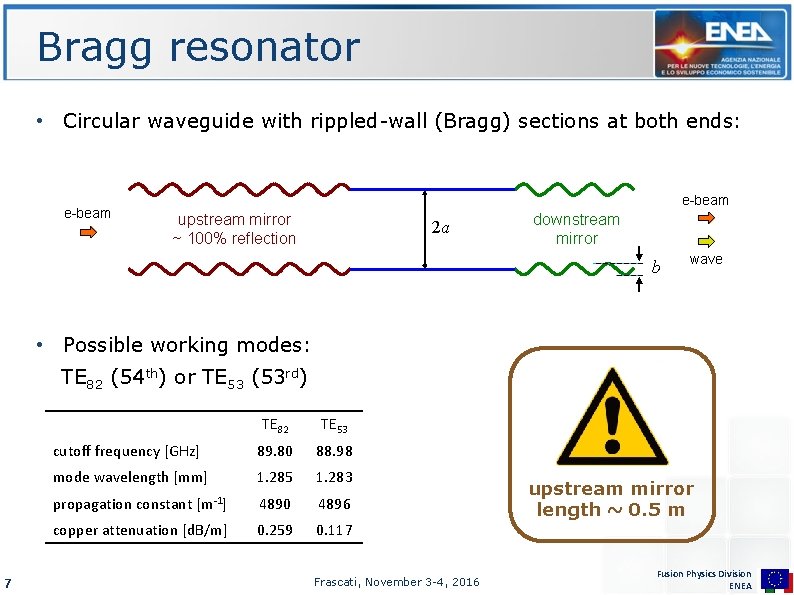
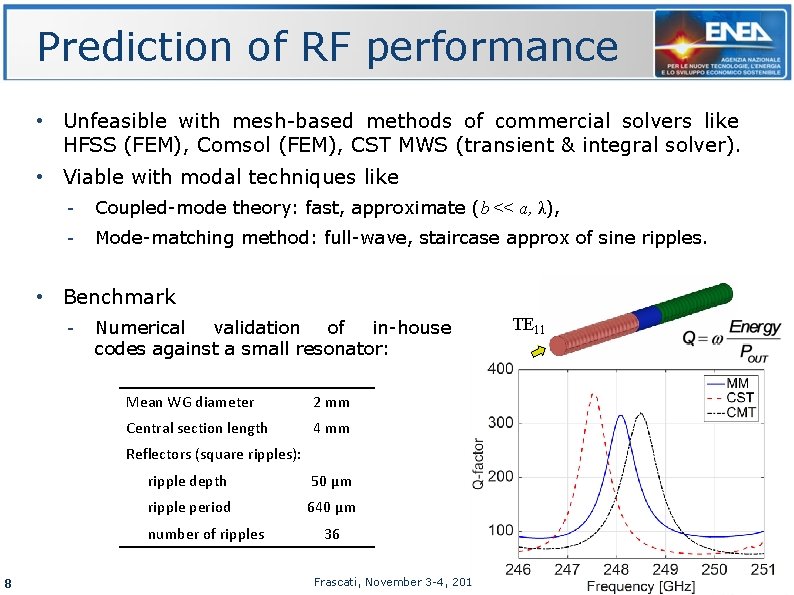
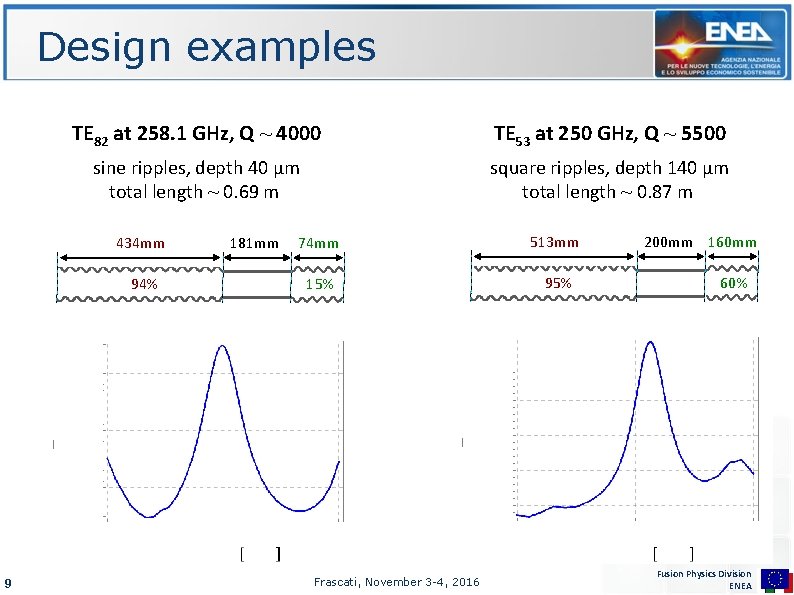
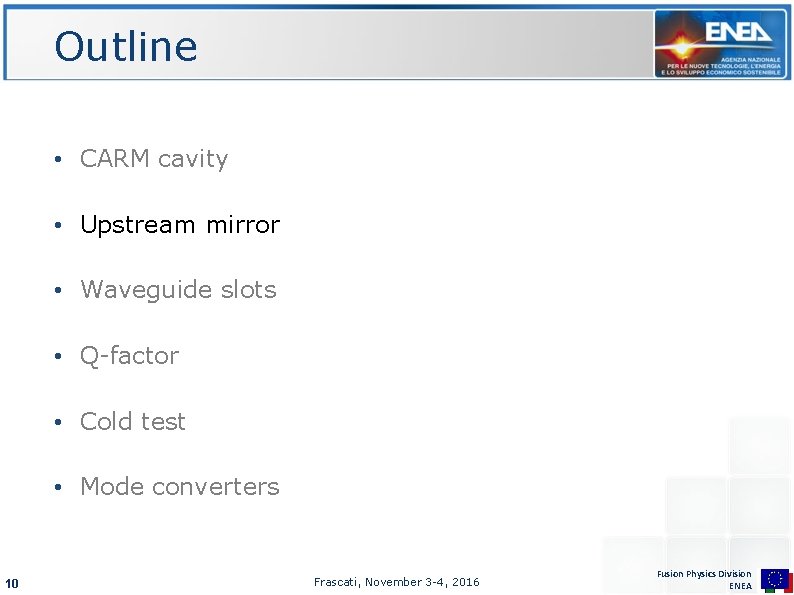
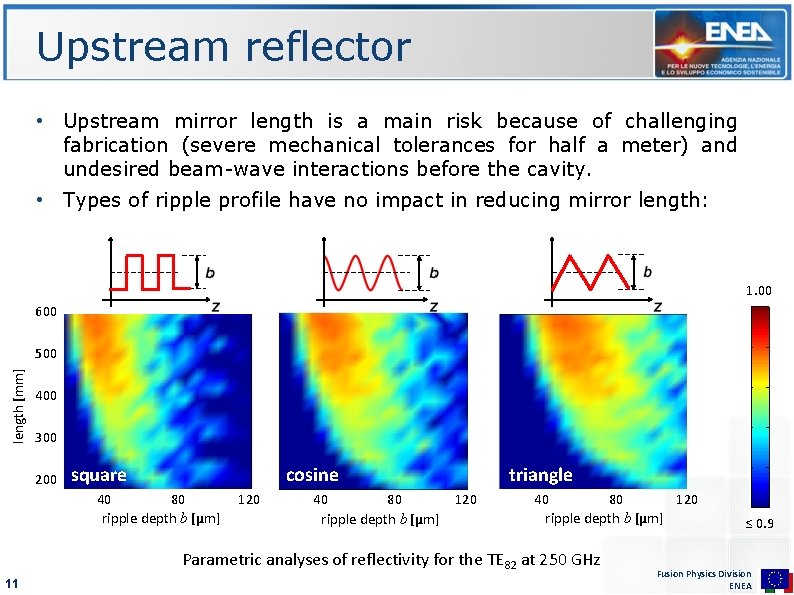
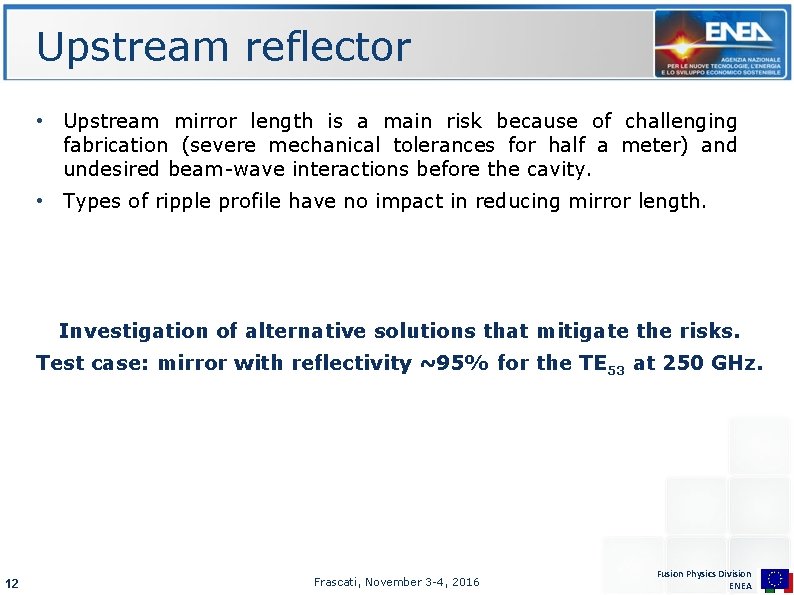
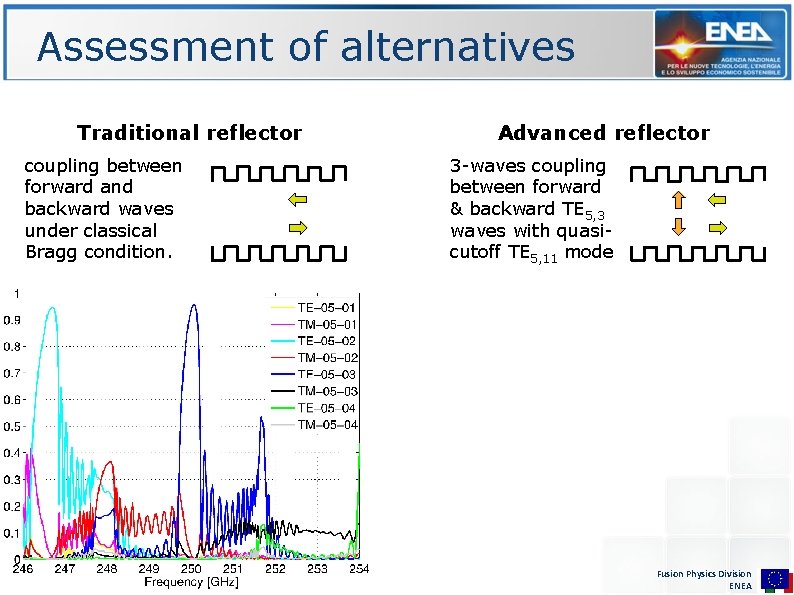
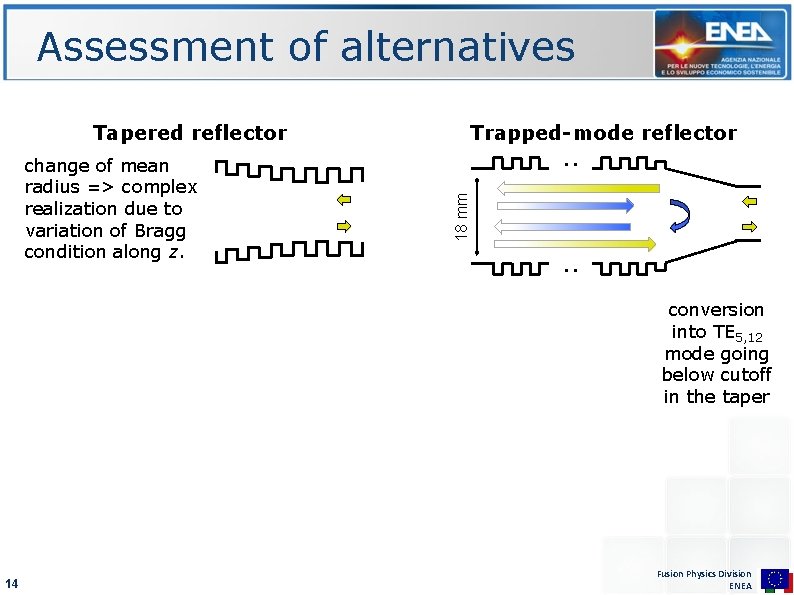
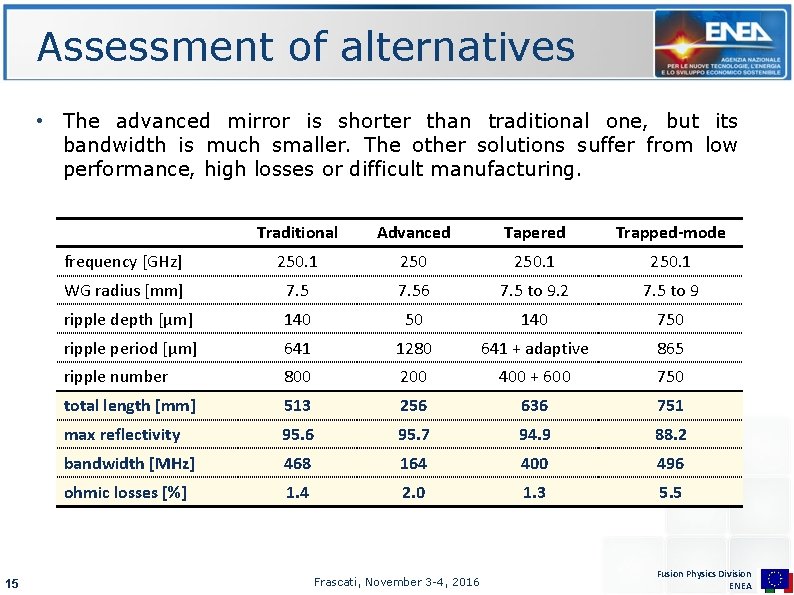
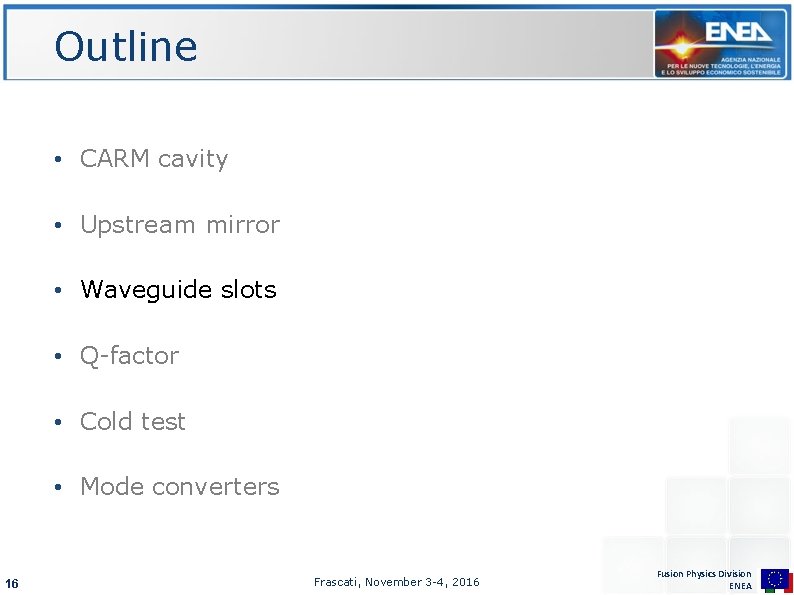
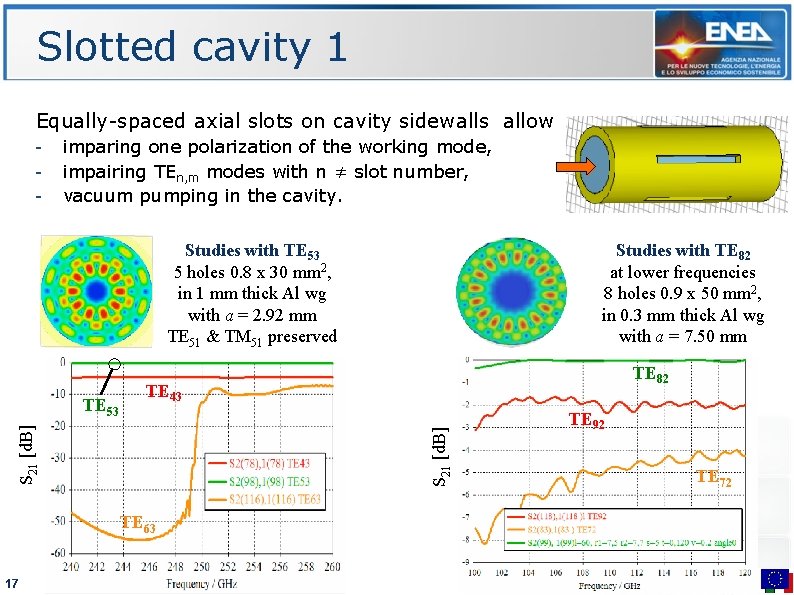
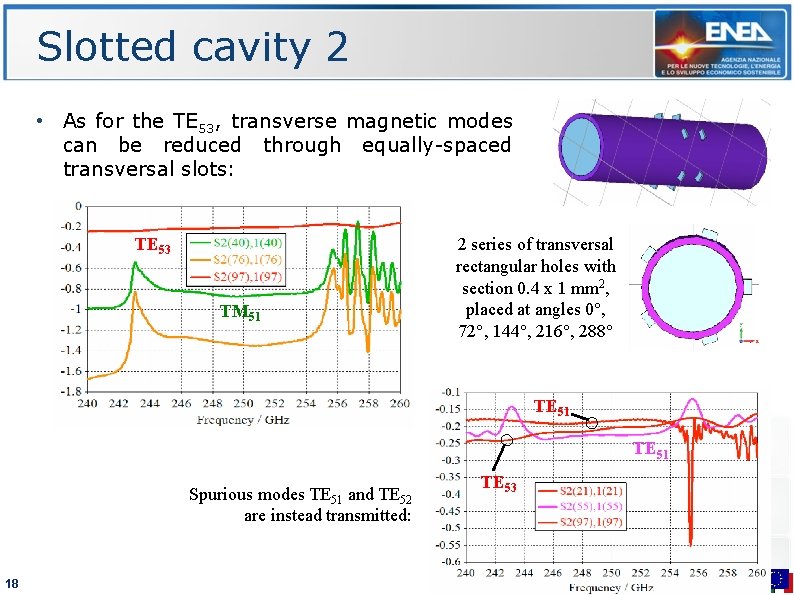
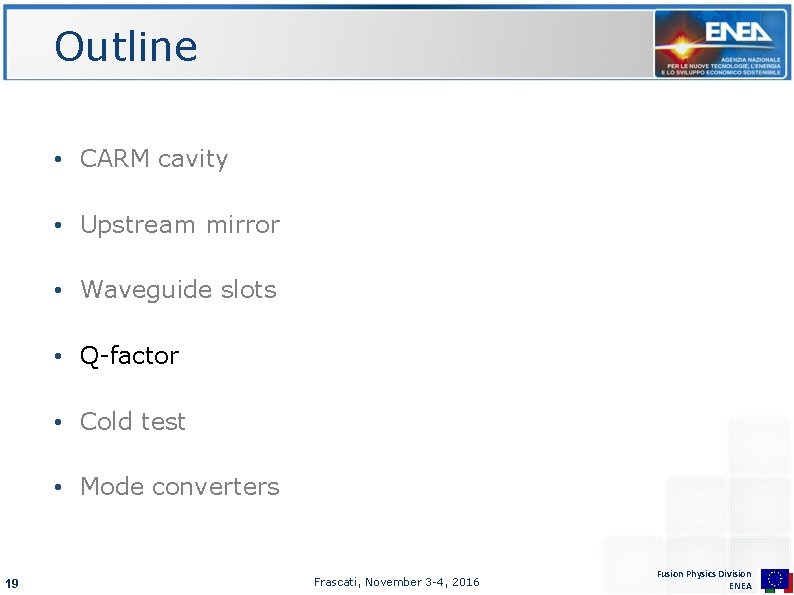
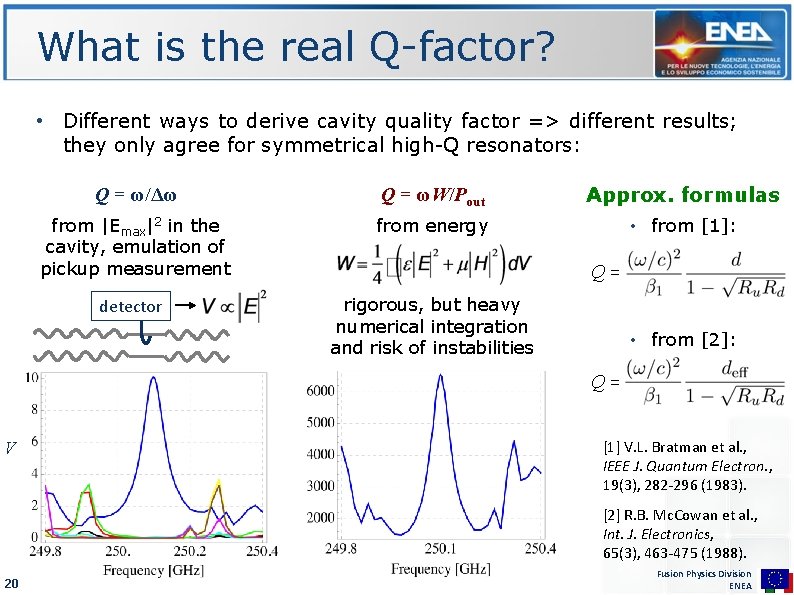
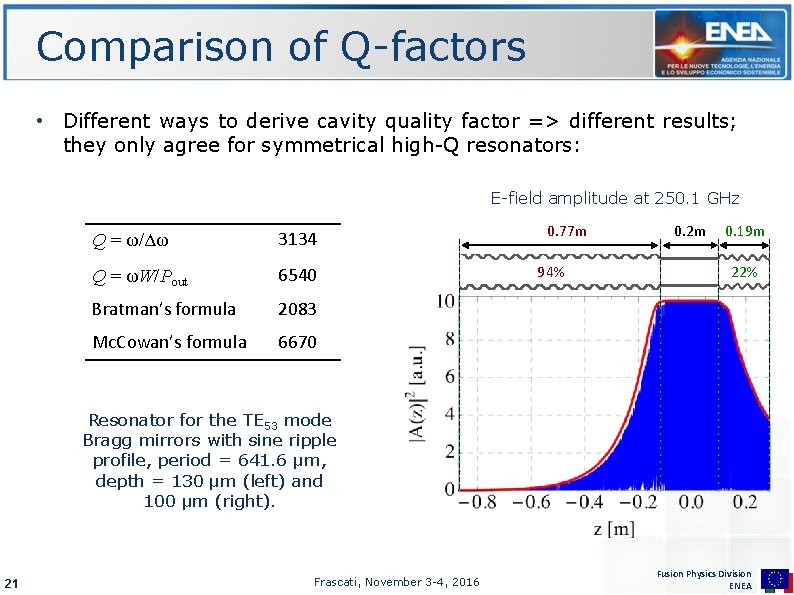
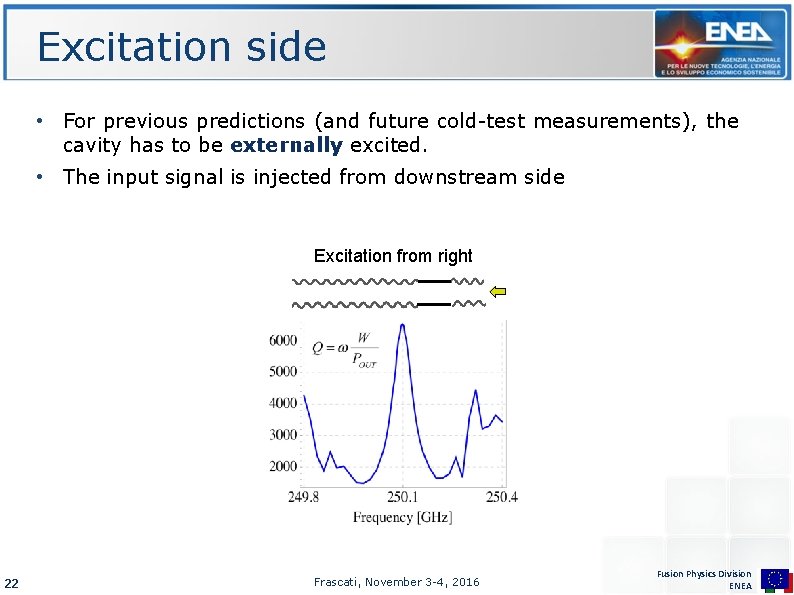
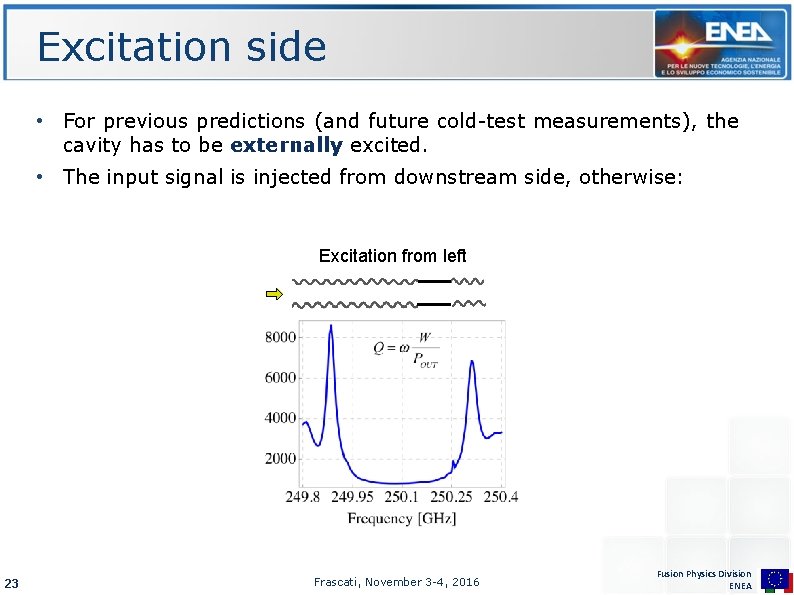
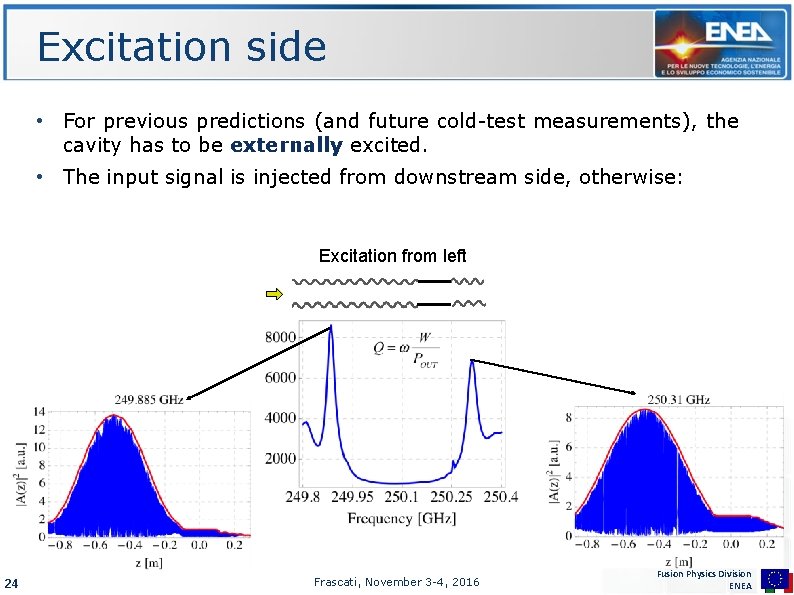
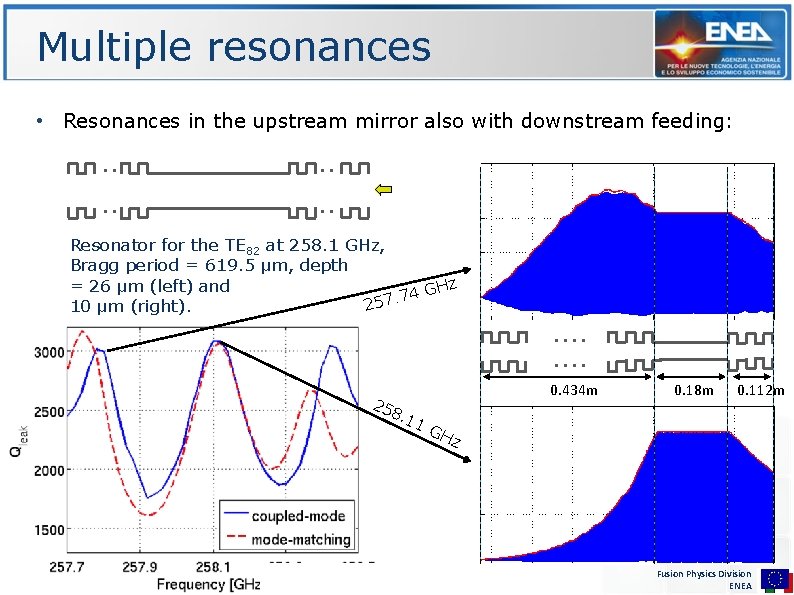
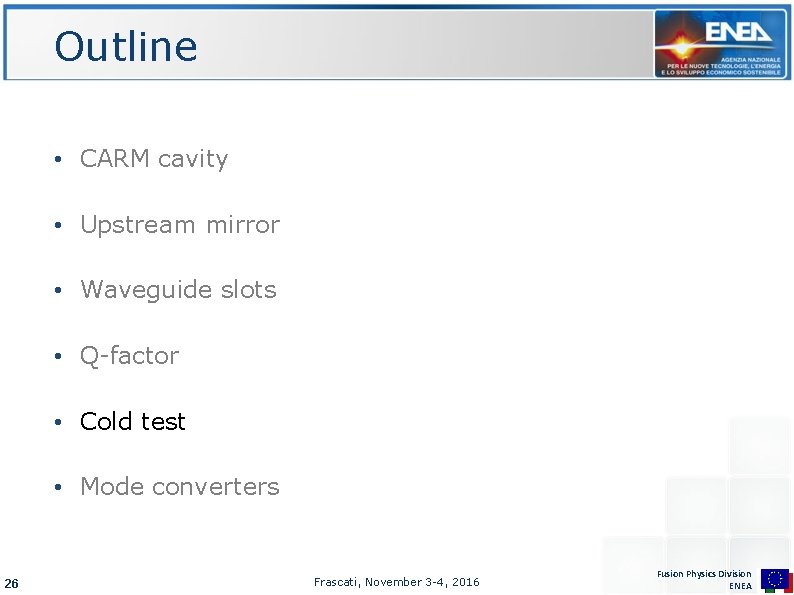
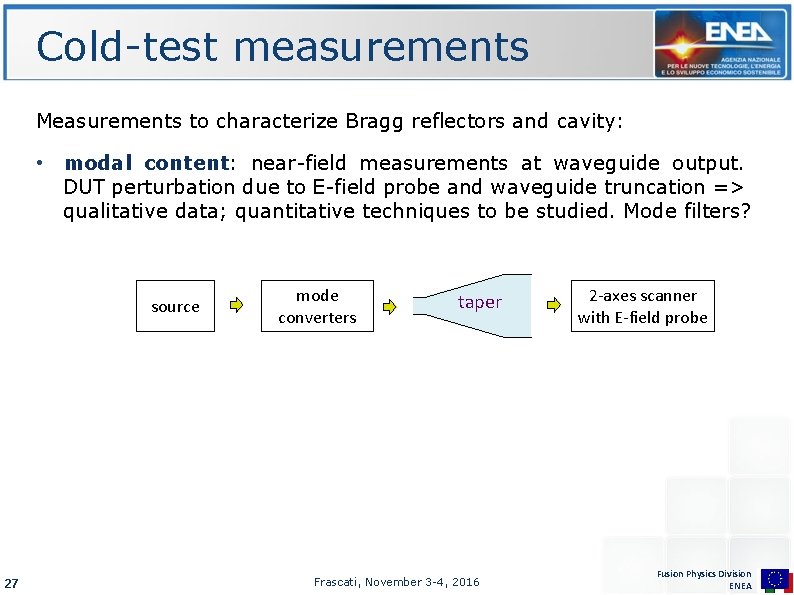
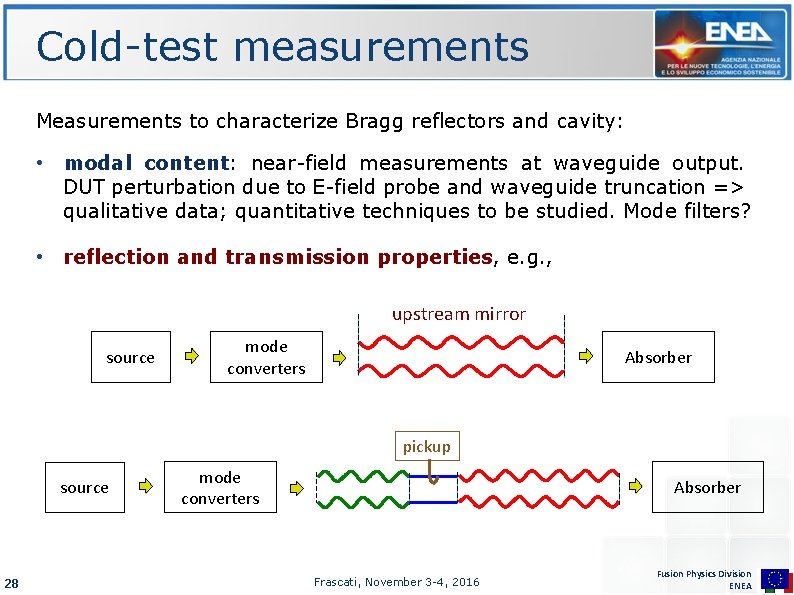
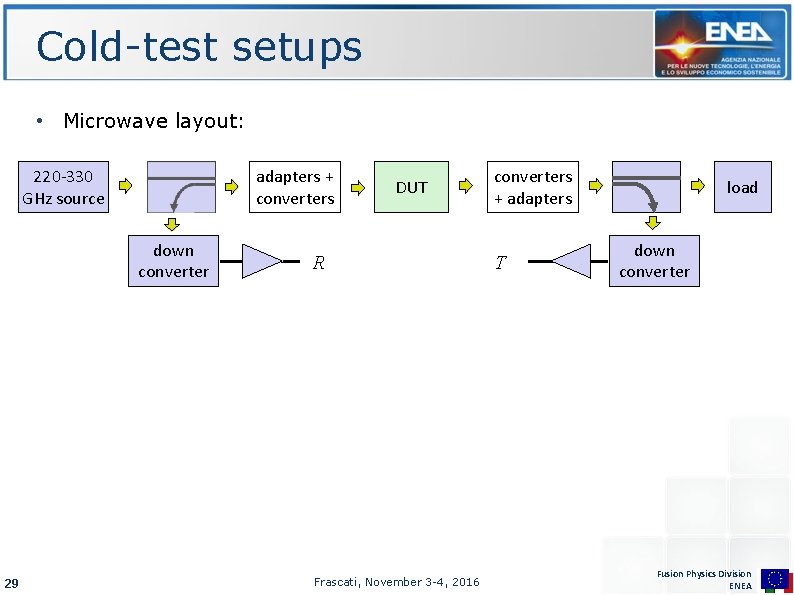
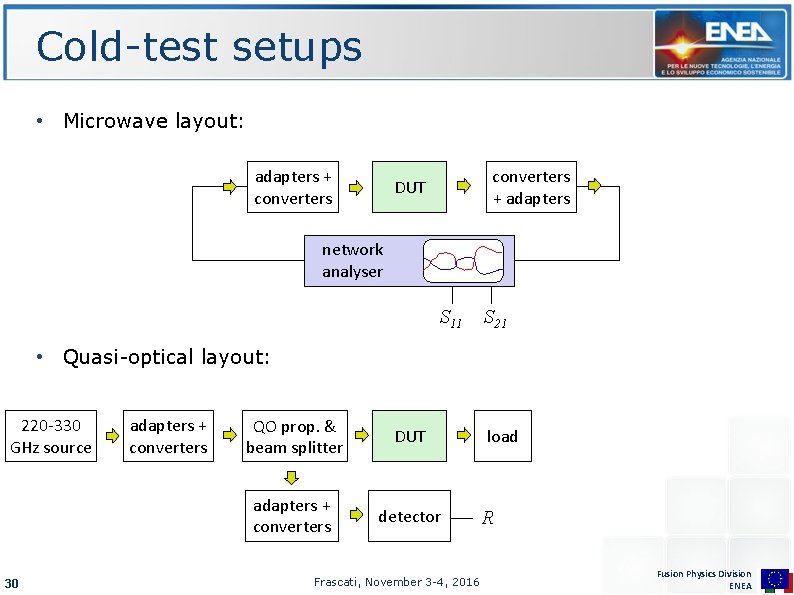
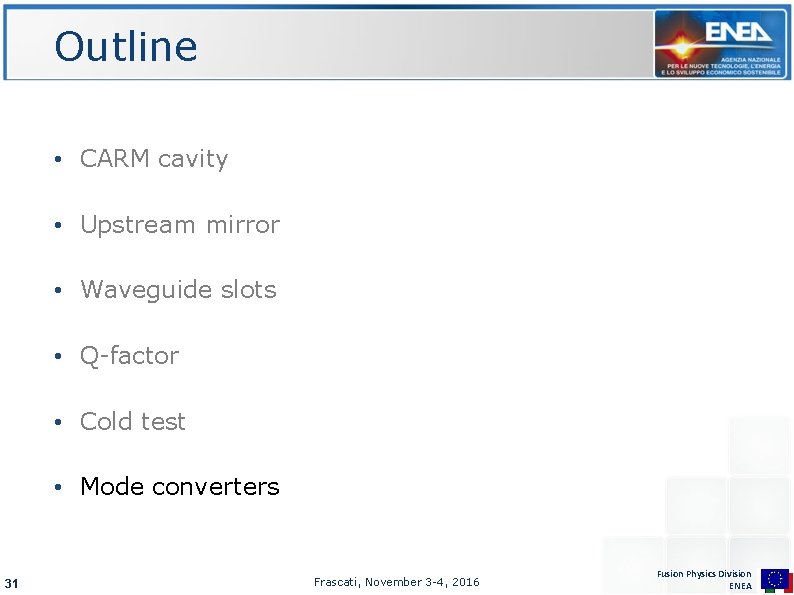
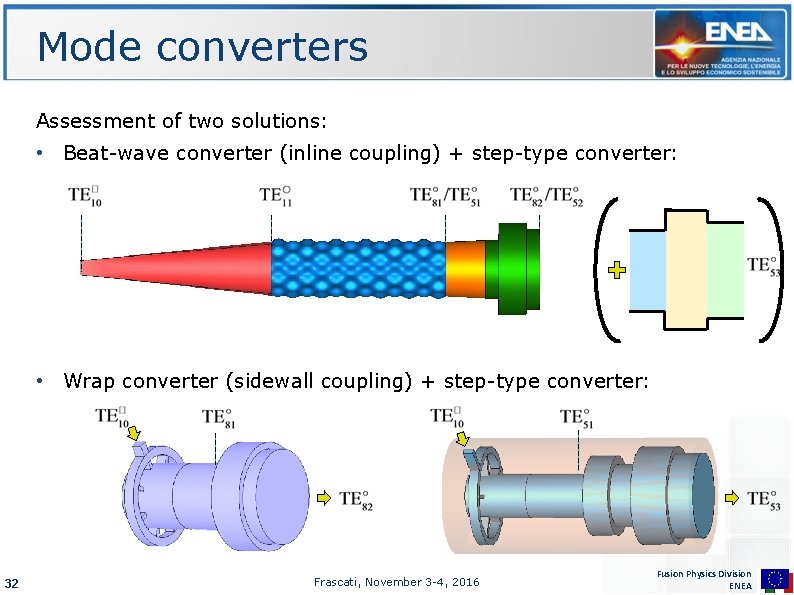
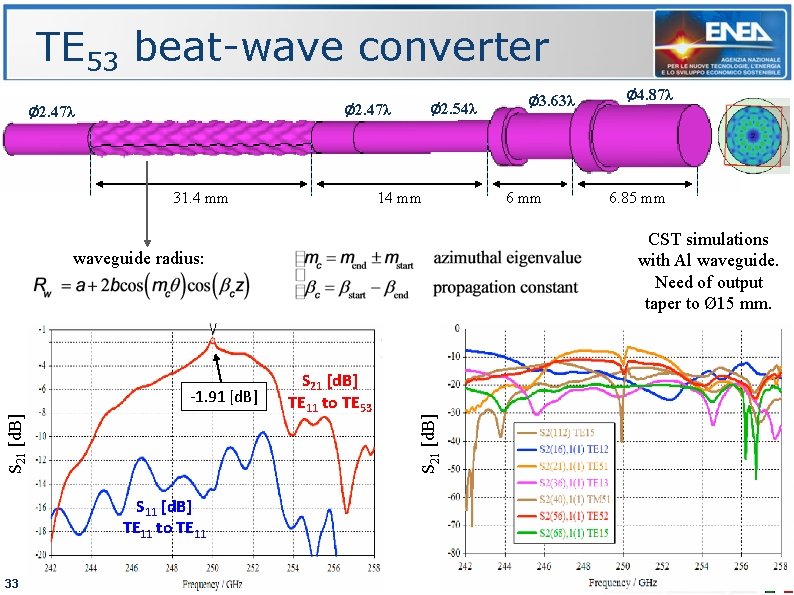
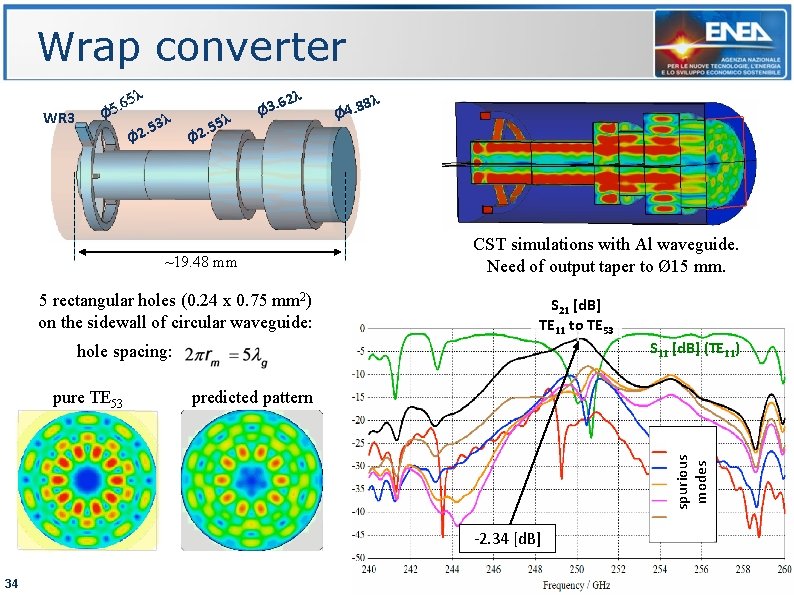
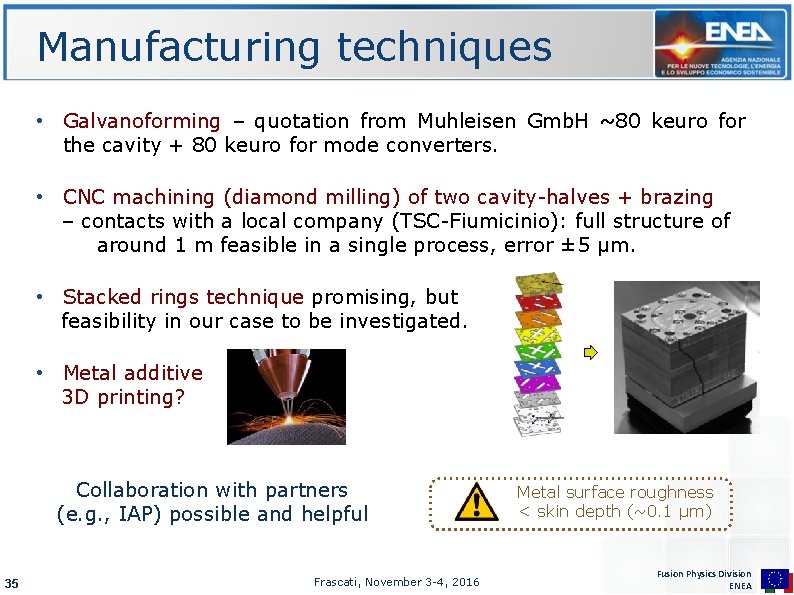
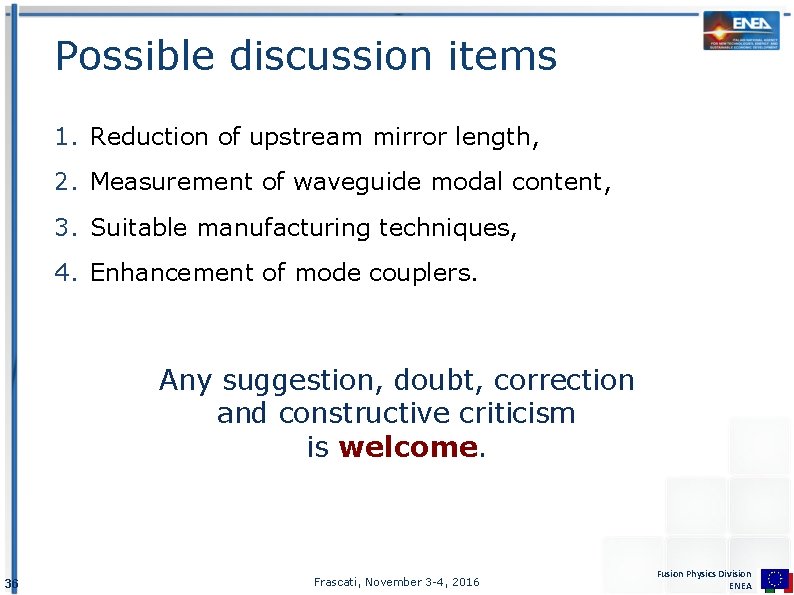
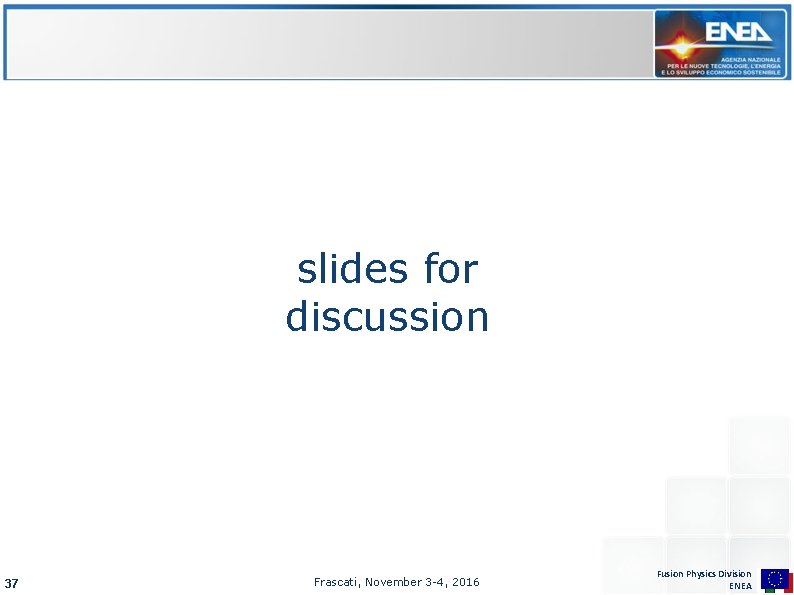
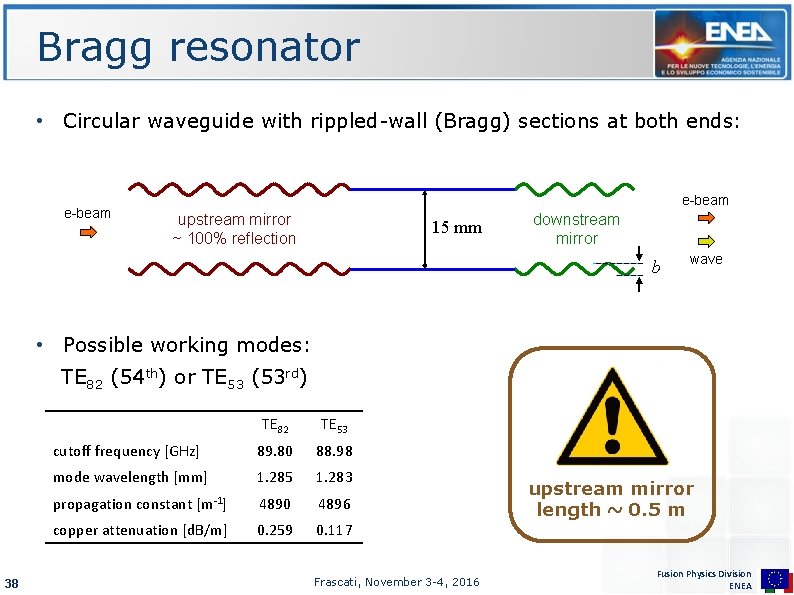
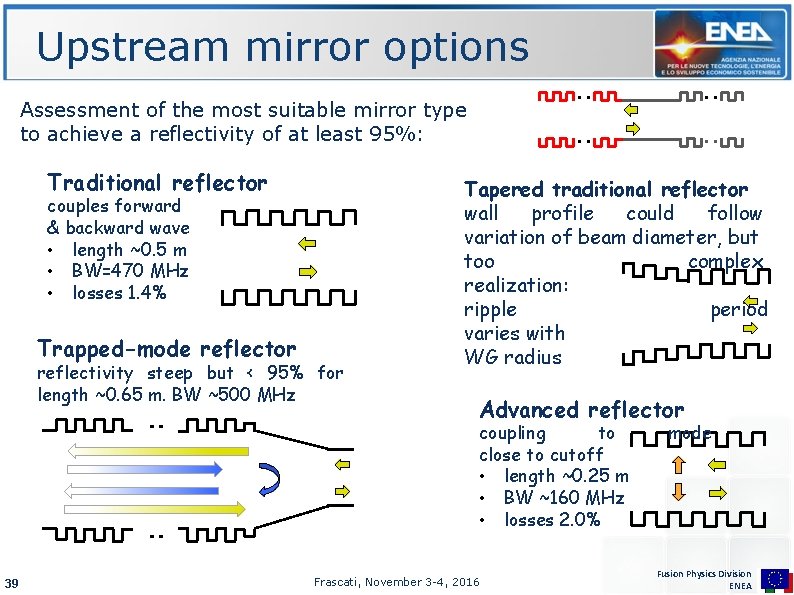
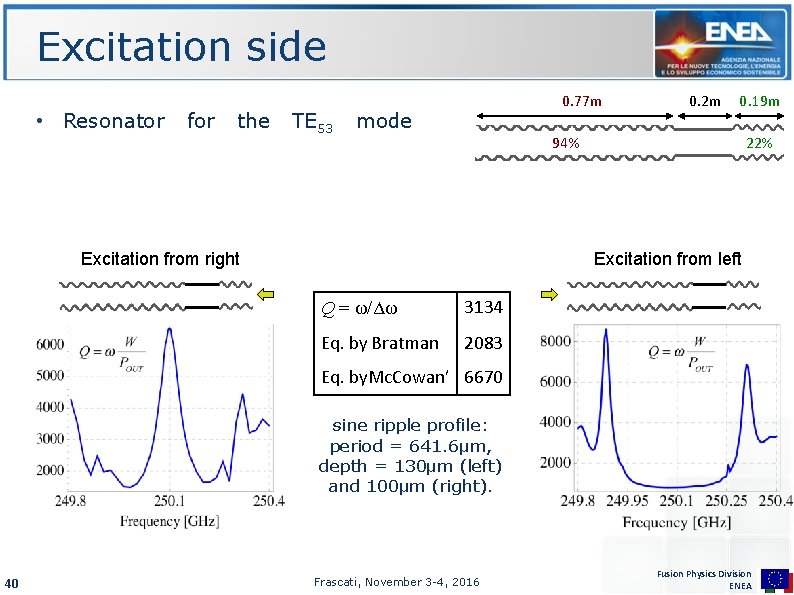
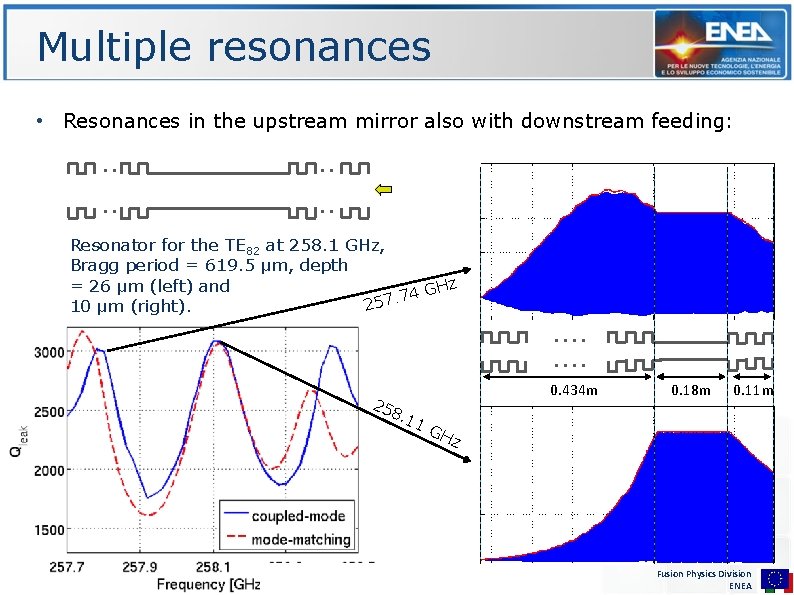
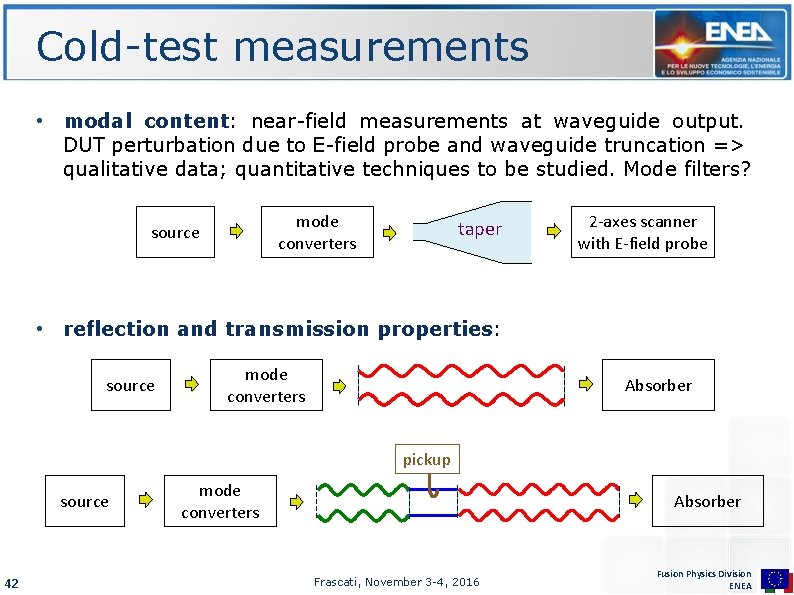
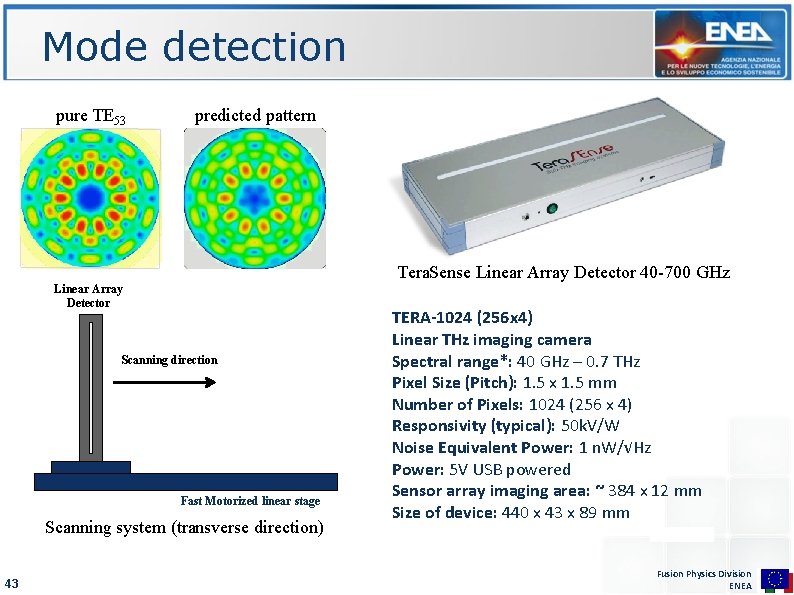
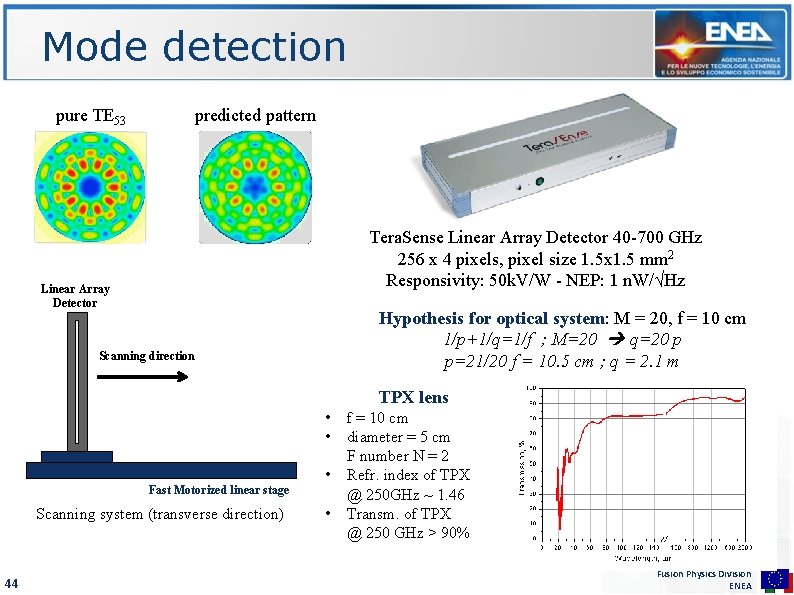
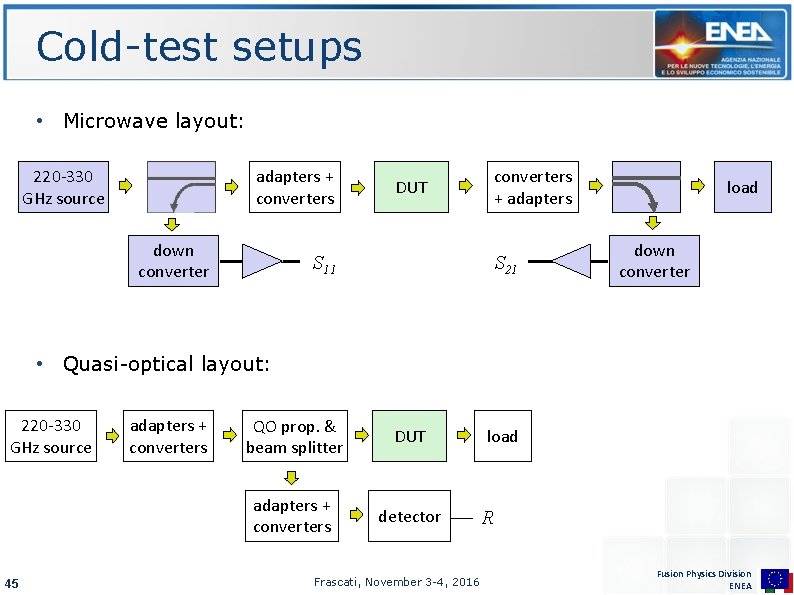
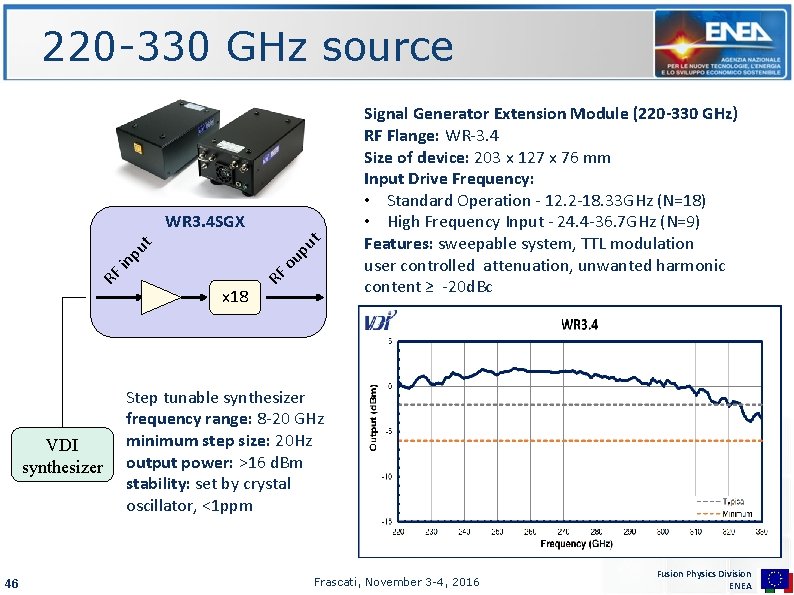
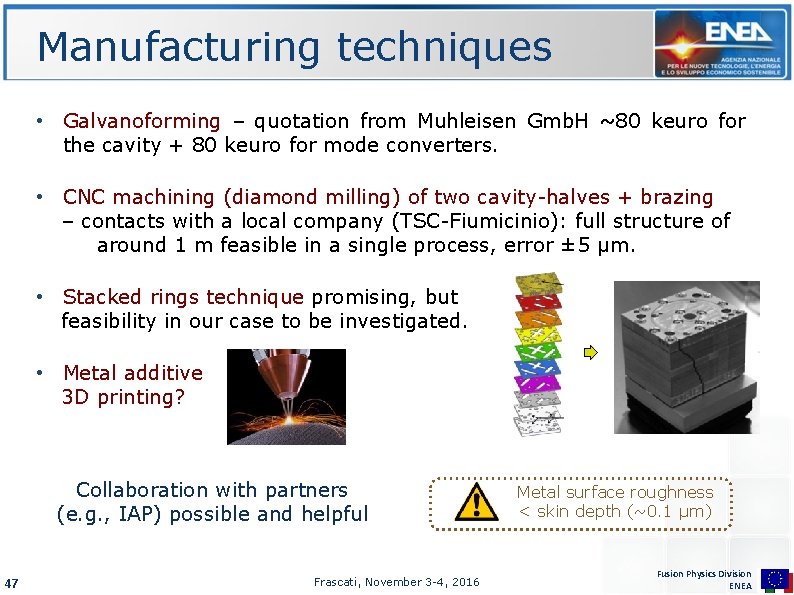
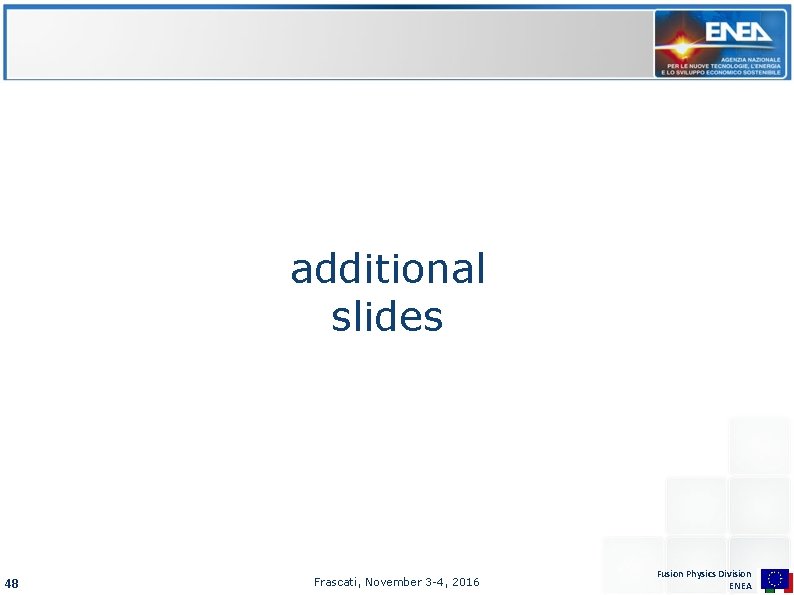
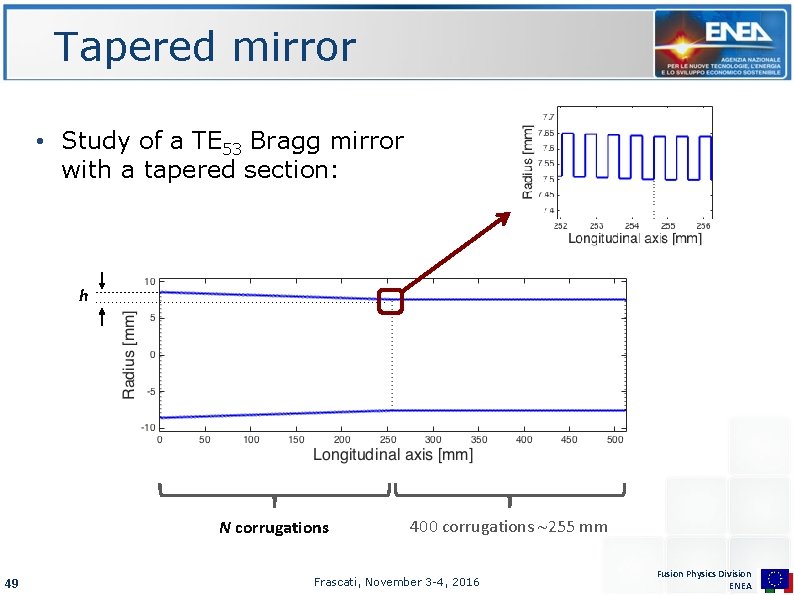
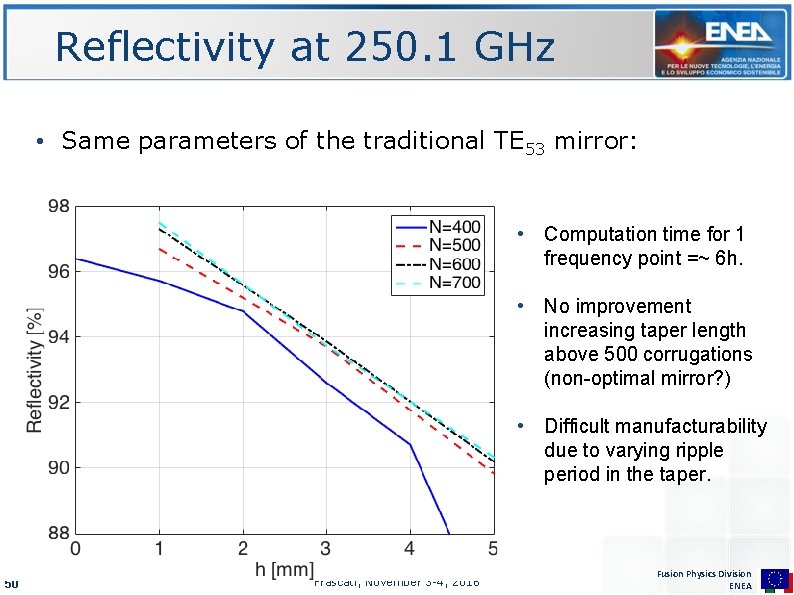
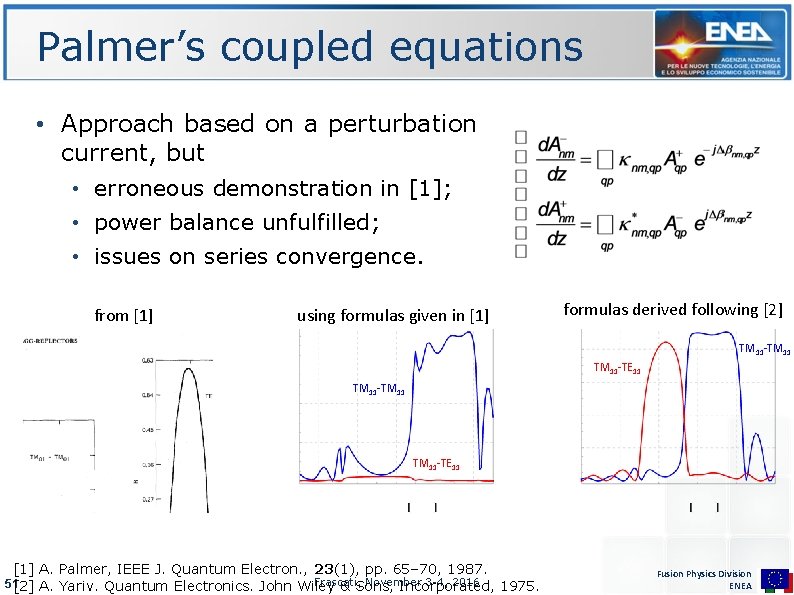
![Solymar’s coupled equations • Widespread approach [1, 2], used by Mc. Cowan, Thumm, … Solymar’s coupled equations • Widespread approach [1, 2], used by Mc. Cowan, Thumm, …](https://slidetodoc.com/presentation_image_h2/9c82b675c66be87ef7f1b4dc394a2855/image-52.jpg)
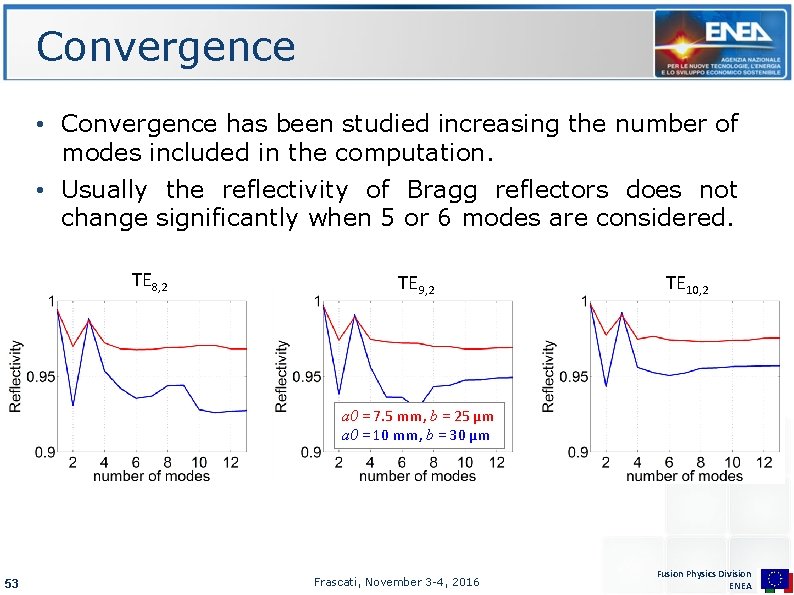
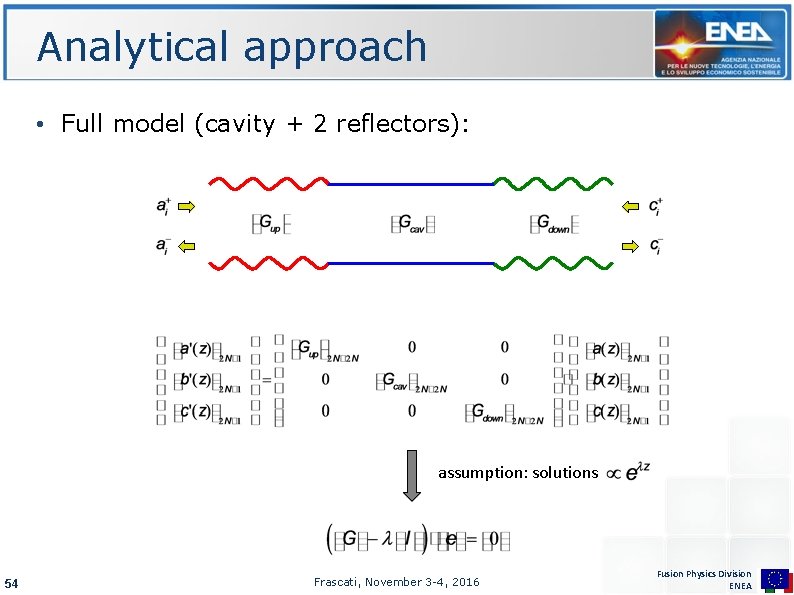
- Slides: 54
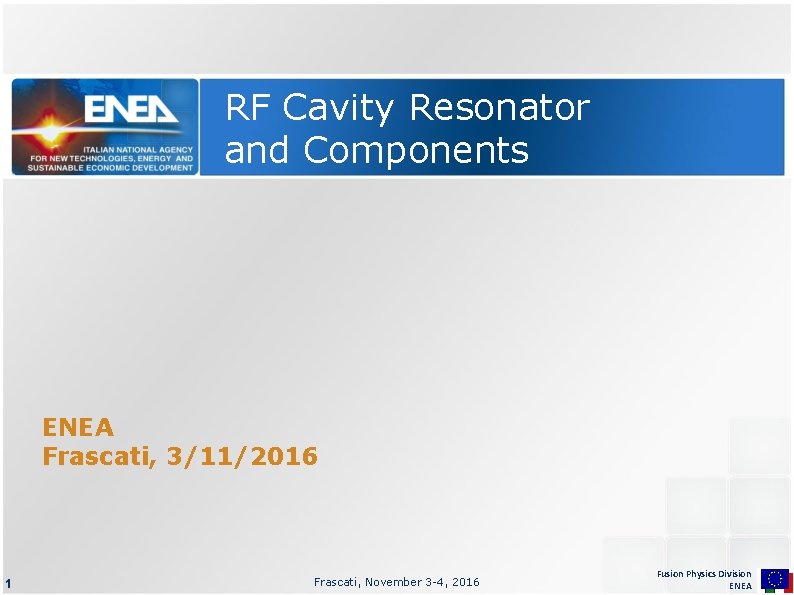
RF Cavity Resonator and Components ENEA Frascati, 3/11/2016 1 Frascati, November 3 -4, 2016 Fusion Physics Division ENEA
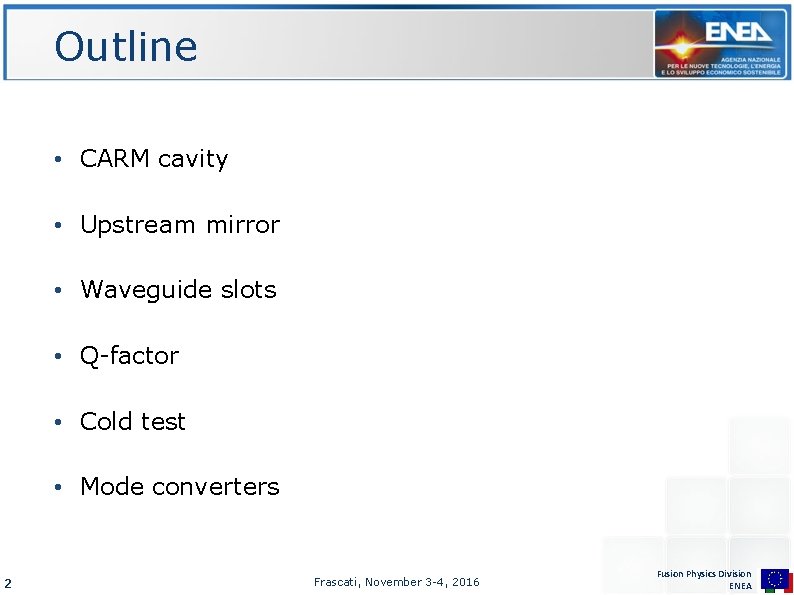
Outline • CARM cavity • Upstream mirror • Waveguide slots • Q-factor • Cold test • Mode converters 2 Frascati, November 3 -4, 2016 Fusion Physics Division ENEA
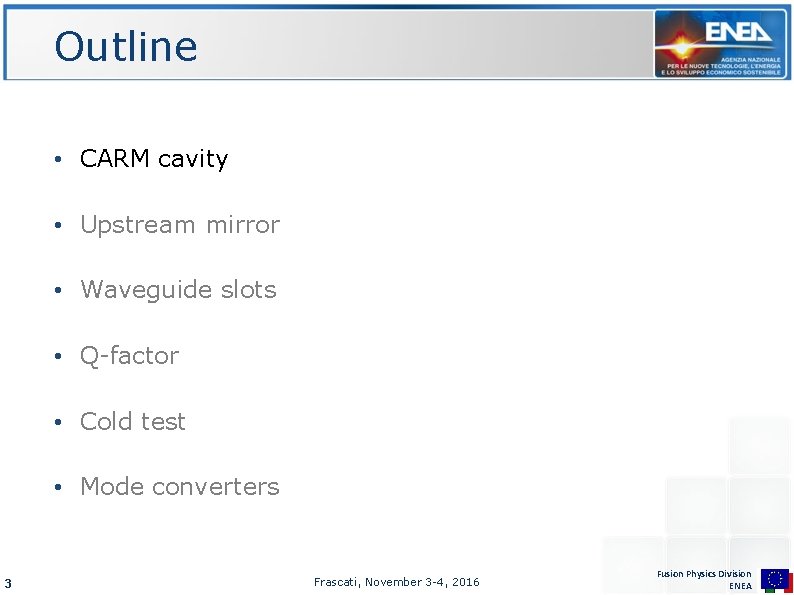
Outline • CARM cavity • Upstream mirror • Waveguide slots • Q-factor • Cold test • Mode converters 3 Frascati, November 3 -4, 2016 Fusion Physics Division ENEA
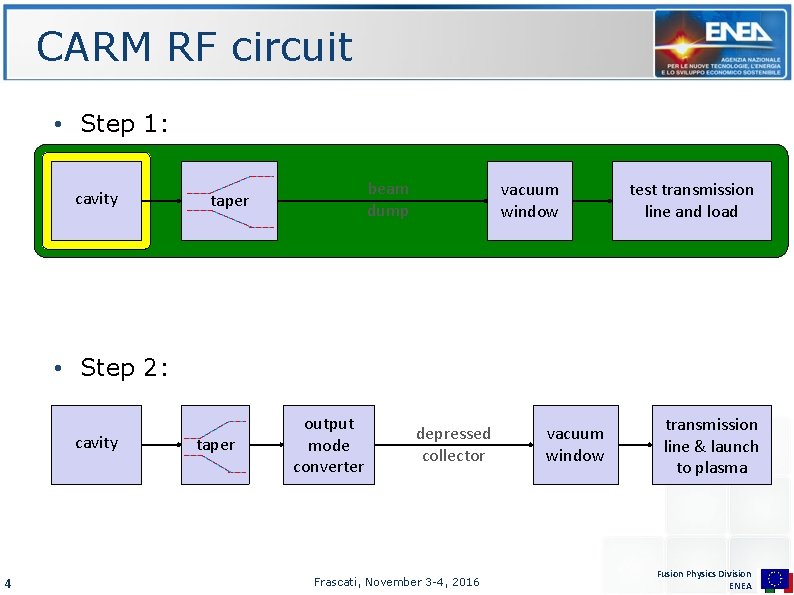
CARM RF circuit • Step 1: cavity beam dump taper vacuum window test transmission line and load • Step 2: cavity 4 taper output mode converter depressed collector Frascati, November 3 -4, 2016 vacuum window transmission line & launch to plasma Fusion Physics Division ENEA
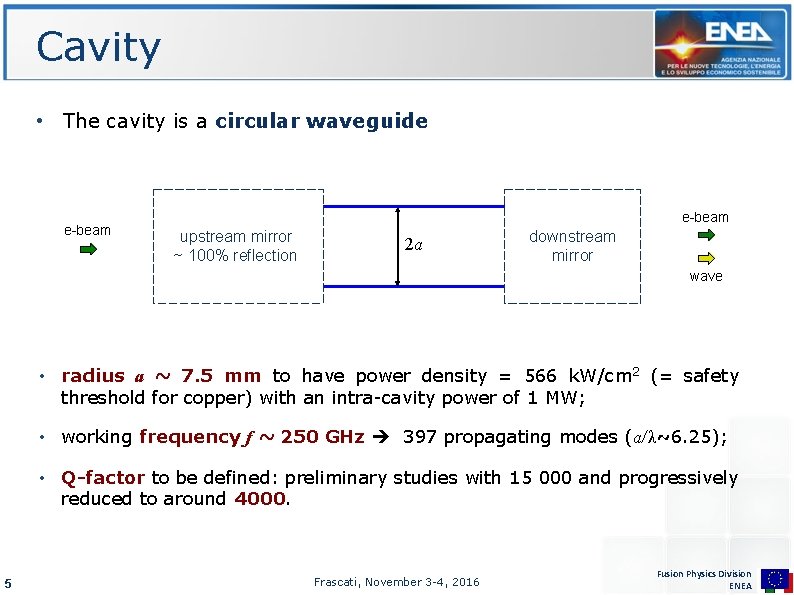
Cavity • The cavity is a circular waveguide e-beam upstream mirror ~ 100% reflection 2 a downstream mirror wave • radius a ~ 7. 5 mm to have power density = 566 k. W/cm 2 (= safety threshold for copper) with an intra-cavity power of 1 MW; • working frequency f ~ 250 GHz 397 propagating modes (a/λ~6. 25); • Q-factor to be defined: preliminary studies with 15 000 and progressively reduced to around 4000. 5 Frascati, November 3 -4, 2016 Fusion Physics Division ENEA
![Cavity options Quasioptical resonator 1 high thermal load on discrete mirrors Bragg Cavity options • Quasi-optical resonator [1]: high thermal load on discrete mirrors • Bragg](https://slidetodoc.com/presentation_image_h2/9c82b675c66be87ef7f1b4dc394a2855/image-6.jpg)
Cavity options • Quasi-optical resonator [1]: high thermal load on discrete mirrors • Bragg resonator [2]: e-beam ohmic losses spread on distributed mirrors wave [1] T. G. A. Verhoeven et al. , IEEE Trans. on Plasma Sci. , 27(4), 1084 -1091 (1999). Frascati, 2016 6[2] V. L. Bratman et al. , IEEE J. Quantum Electron. , 19(3), November 282 -296 3 -4, (1983). Fusion Physics Division ENEA
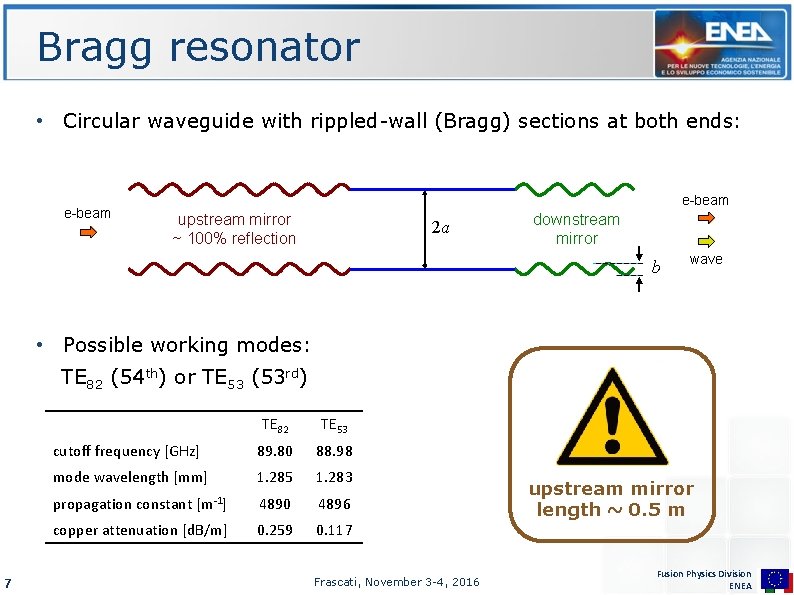
Bragg resonator • Circular waveguide with rippled-wall (Bragg) sections at both ends: e-beam upstream mirror ~ 100% reflection 2 a downstream mirror b wave • Possible working modes: TE 82 (54 th) or TE 53 (53 rd) TE 82 TE 53 cutoff frequency [GHz] 89. 80 88. 98 mode wavelength [mm] 1. 285 1. 283 [m-1] 4890 4896 copper attenuation [d. B/m] 0. 259 0. 117 propagation constant 7 Frascati, November 3 -4, 2016 upstream mirror length ~ 0. 5 m Fusion Physics Division ENEA
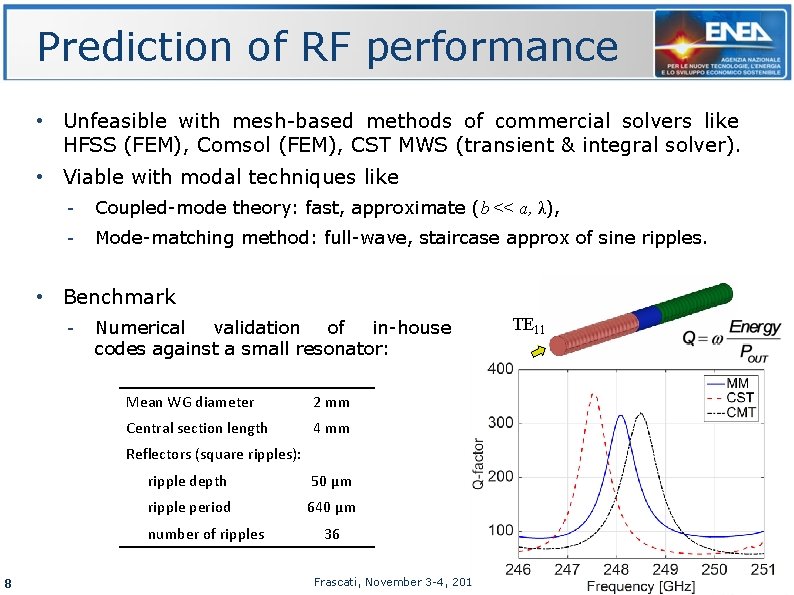
Prediction of RF performance • Unfeasible with mesh-based methods of commercial solvers like HFSS (FEM), Comsol (FEM), CST MWS (transient & integral solver). • Viable with modal techniques like - Coupled-mode theory: fast, approximate (b << a, λ), - Mode-matching method: full-wave, staircase approx of sine ripples. • Benchmark - Numerical validation of in-house codes against a small resonator: Mean WG diameter 2 mm Central section length 4 mm TE 11 Reflectors (square ripples): ripple depth 50 μm ripple period 640 μm number of ripples 8 36 Frascati, November 3 -4, 2016 Fusion Physics Division ENEA
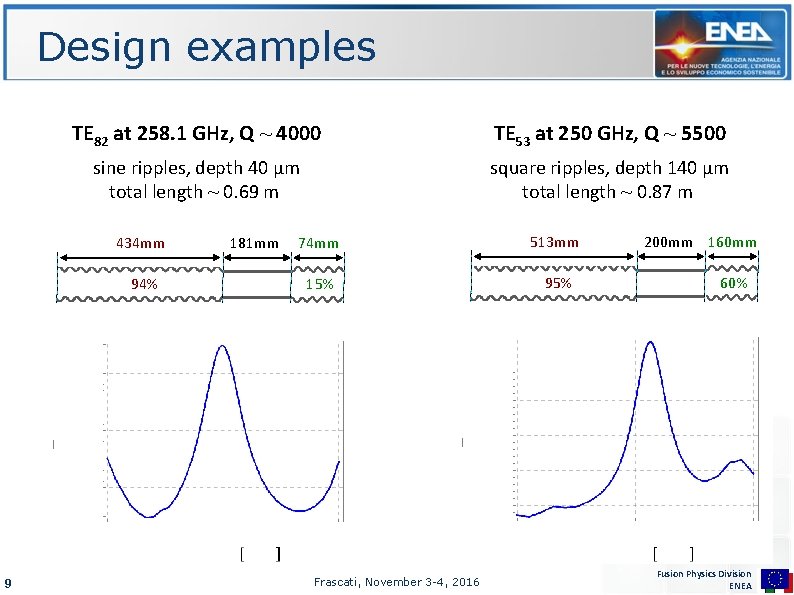
Design examples TE 82 at 258. 1 GHz, Q ~ 4000 TE 53 at 250 GHz, Q ~ 5500 sine ripples, depth 40 μm total length ~ 0. 69 m square ripples, depth 140 μm total length ~ 0. 87 m 434 mm 94% 9 181 mm 74 mm 513 mm 15% 95% Frascati, November 3 -4, 2016 200 mm 160 mm 60% Fusion Physics Division ENEA
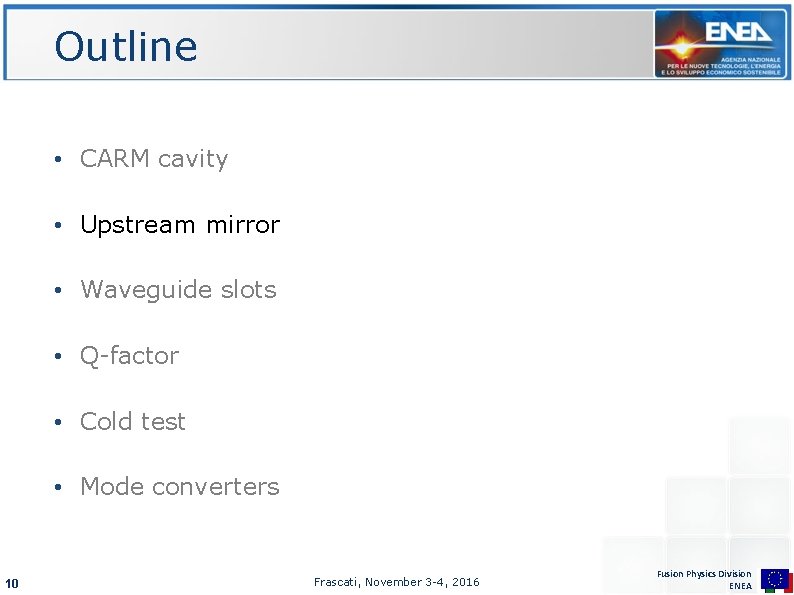
Outline • CARM cavity • Upstream mirror • Waveguide slots • Q-factor • Cold test • Mode converters 10 Frascati, November 3 -4, 2016 Fusion Physics Division ENEA
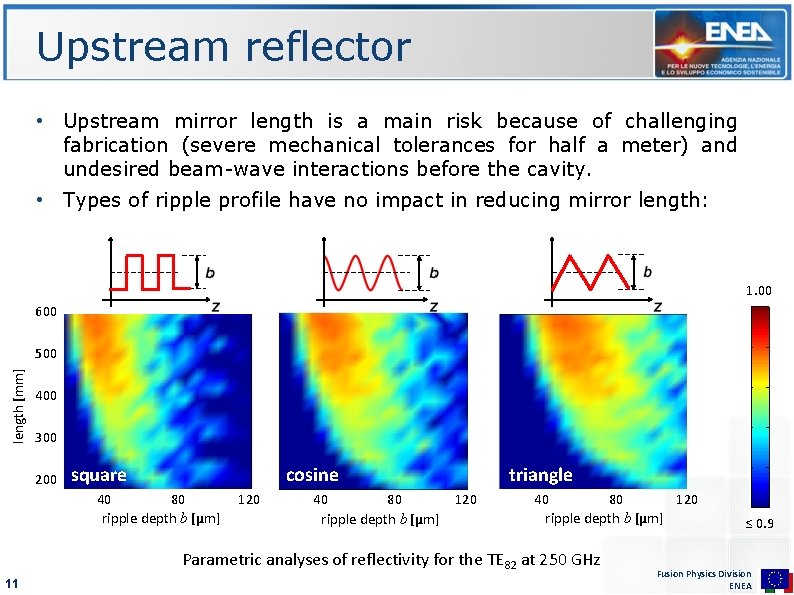
Upstream reflector • Upstream mirror length is a main risk because of challenging fabrication (severe mechanical tolerances for half a meter) and undesired beam-wave interactions before the cavity. • Types of ripple profile have no impact in reducing mirror length: 1. 00 600 length [mm] 500 400 300 200 square cosine 40 80 ripple depth b [μm] 120 40 80 ripple depth b [μm] triangle 120 40 80 120 ripple depth b [μm] Parametric analyses of reflectivity for the TE 82 at 250 GHz 11 Frascati, November 3 -4, 2016 ≤ 0. 9 Fusion Physics Division ENEA
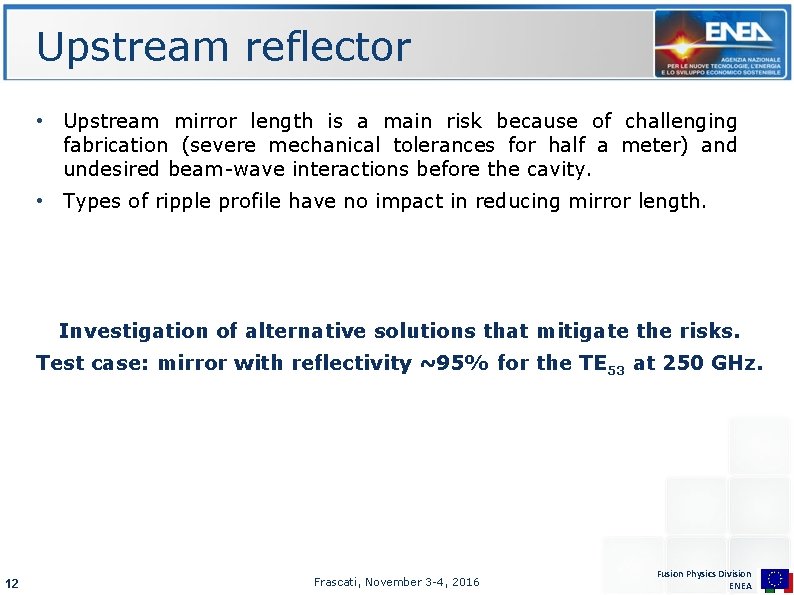
Upstream reflector • Upstream mirror length is a main risk because of challenging fabrication (severe mechanical tolerances for half a meter) and undesired beam-wave interactions before the cavity. • Types of ripple profile have no impact in reducing mirror length. Investigation of alternative solutions that mitigate the risks. Test case: mirror with reflectivity ~95% for the TE 53 at 250 GHz. 12 Frascati, November 3 -4, 2016 Fusion Physics Division ENEA
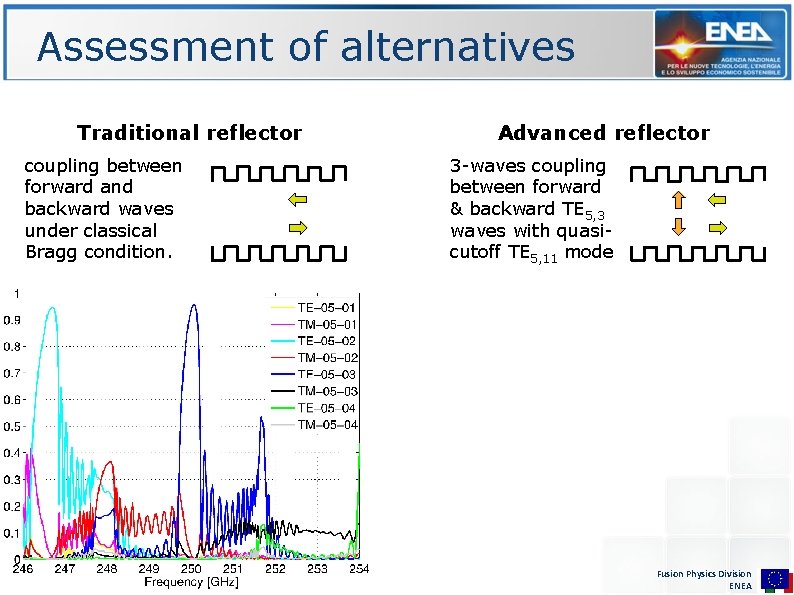
Assessment of alternatives Traditional reflector coupling between forward and backward waves under classical Bragg condition. 13 Advanced reflector 3 -waves coupling between forward & backward TE 5, 3 waves with quasicutoff TE 5, 11 mode Frascati, November 3 -4, 2016 Fusion Physics Division ENEA
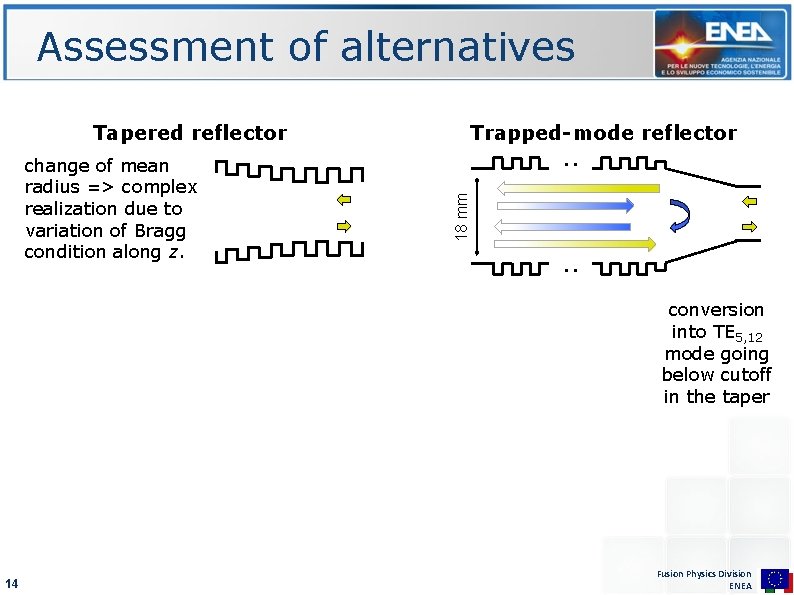
Assessment of alternatives change of mean radius => complex realization due to variation of Bragg condition along z. Trapped-mode reflector 18 mm Tapered reflector conversion into TE 5, 12 mode going below cutoff in the taper 14 Frascati, November 3 -4, 2016 Fusion Physics Division ENEA
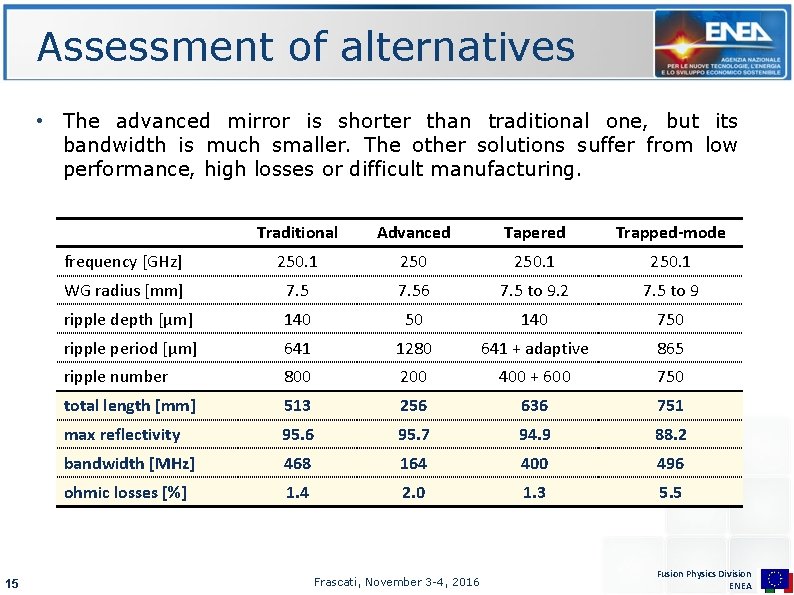
Assessment of alternatives • The advanced mirror is shorter than traditional one, but its bandwidth is much smaller. The other solutions suffer from low performance, high losses or difficult manufacturing. 15 Traditional Advanced Tapered Trapped-mode frequency [GHz] 250. 1 WG radius [mm] 7. 56 7. 5 to 9. 2 7. 5 to 9 ripple depth [μm] 140 50 140 750 ripple period [μm] 641 1280 641 + adaptive 865 ripple number 800 200 400 + 600 750 total length [mm] 513 256 636 751 max reflectivity 95. 6 95. 7 94. 9 88. 2 bandwidth [MHz] 468 164 400 496 ohmic losses [%] 1. 4 2. 0 1. 3 5. 5 Frascati, November 3 -4, 2016 Fusion Physics Division ENEA
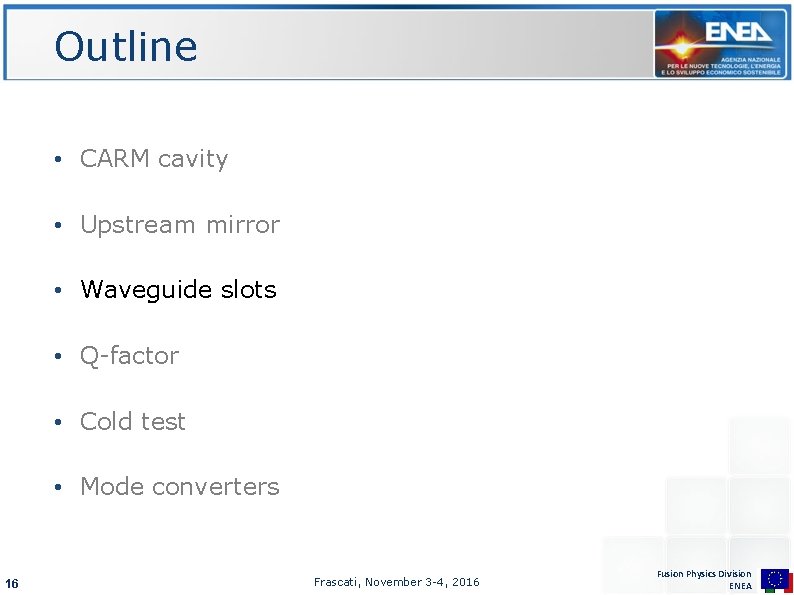
Outline • CARM cavity • Upstream mirror • Waveguide slots • Q-factor • Cold test • Mode converters 16 Frascati, November 3 -4, 2016 Fusion Physics Division ENEA
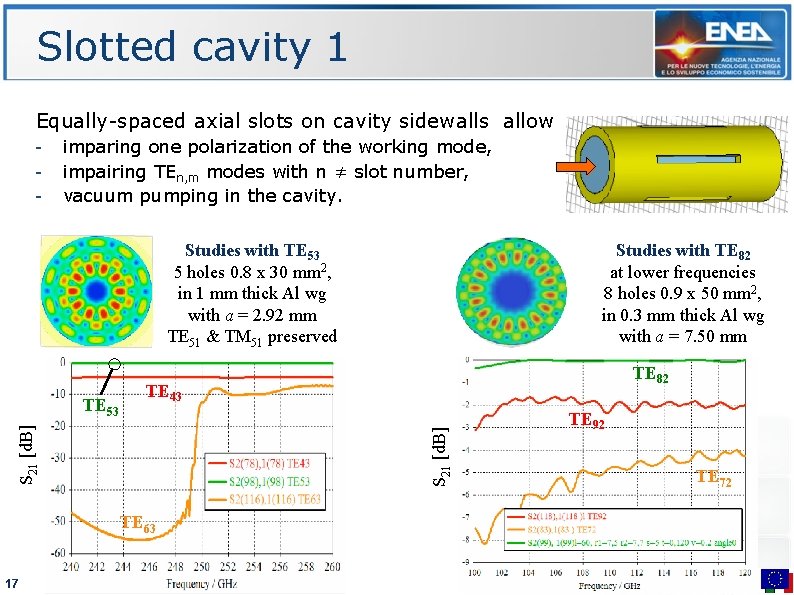
Slotted cavity 1 Equally-spaced axial slots on cavity sidewalls allow - imparing one polarization of the working mode, impairing TEn, m modes with n ≠ slot number, vacuum pumping in the cavity. Studies with TE 53 5 holes 0. 8 x 30 mm 2, in 1 mm thick Al wg with a = 2. 92 mm TE 51 & TM 51 preserved TE 82 TE 43 S 21 [d. B] TE 53 Studies with TE 82 at lower frequencies 8 holes 0. 9 x 50 mm 2, in 0. 3 mm thick Al wg with a = 7. 50 mm TE 92 TE 72 TE 63 17 Frascati, November 3 -4, 2016 Fusion Physics Division ENEA
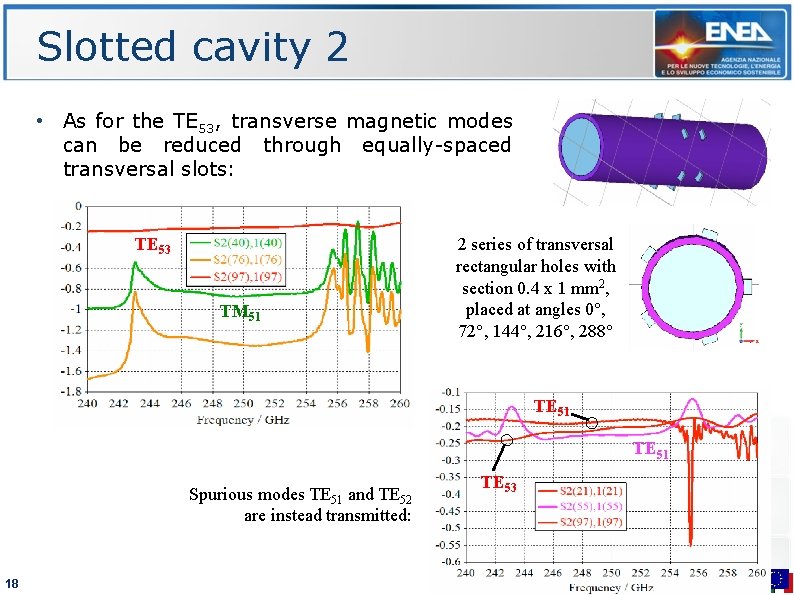
Slotted cavity 2 • As for the TE 53, transverse magnetic modes can be reduced through equally-spaced transversal slots: TE 53 2 series of transversal rectangular holes with section 0. 4 x 1 mm 2, placed at angles 0°, 72°, 144°, 216°, 288° TM 51 TE 51 Spurious modes TE 51 and TE 52 are instead transmitted: 18 Frascati, November 3 -4, 2016 TE 53 Fusion Physics Division ENEA
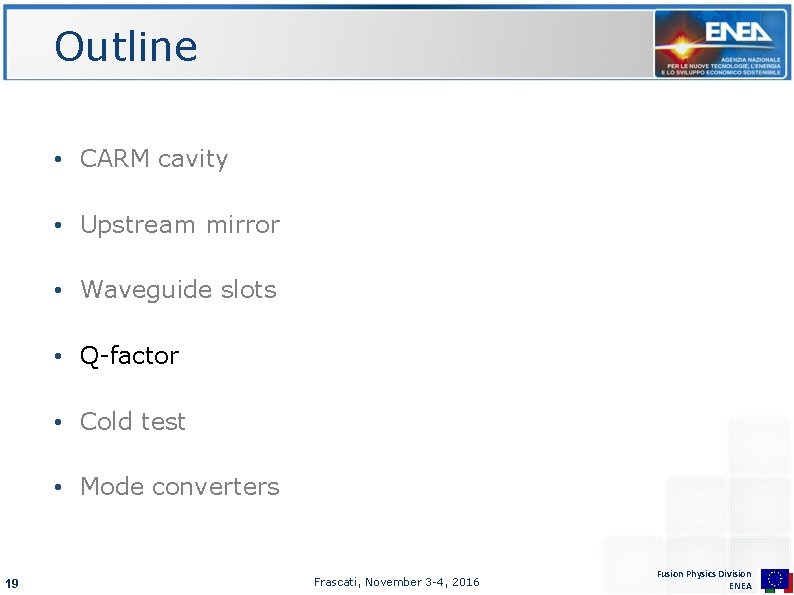
Outline • CARM cavity • Upstream mirror • Waveguide slots • Q-factor • Cold test • Mode converters 19 Frascati, November 3 -4, 2016 Fusion Physics Division ENEA
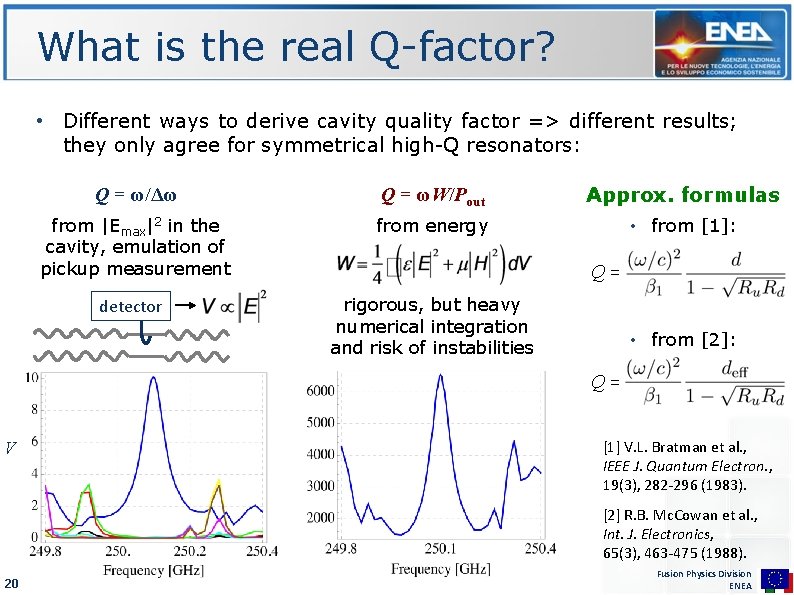
What is the real Q-factor? • Different ways to derive cavity quality factor => different results; they only agree for symmetrical high-Q resonators: Q = ω/Δω Q = ωW/Pout Approx. formulas from |Emax|2 in the cavity, emulation of pickup measurement from energy • from [1]: detector Q= rigorous, but heavy numerical integration and risk of instabilities • from [2]: Q= [1] V. L. Bratman et al. , IEEE J. Quantum Electron. , 19(3), 282 -296 (1983). V [2] R. B. Mc. Cowan et al. , Int. J. Electronics, 65(3), 463 -475 (1988). 20 Frascati, November 3 -4, 2016 Fusion Physics Division ENEA
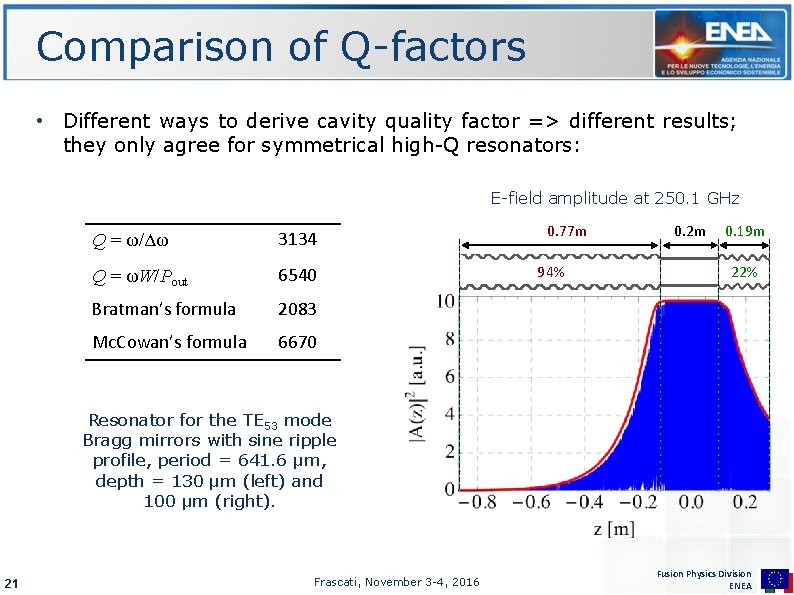
Comparison of Q-factors • Different ways to derive cavity quality factor => different results; they only agree for symmetrical high-Q resonators: E-field amplitude at 250. 1 GHz Q = ω/Δω 3134 Q = ωW/Pout 6540 Bratman’s formula 2083 Mc. Cowan’s formula 6670 0. 77 m 94% 0. 2 m 0. 19 m 22% Resonator for the TE 53 mode Bragg mirrors with sine ripple profile, period = 641. 6 μm, depth = 130 μm (left) and 100 μm (right). 21 Frascati, November 3 -4, 2016 Fusion Physics Division ENEA
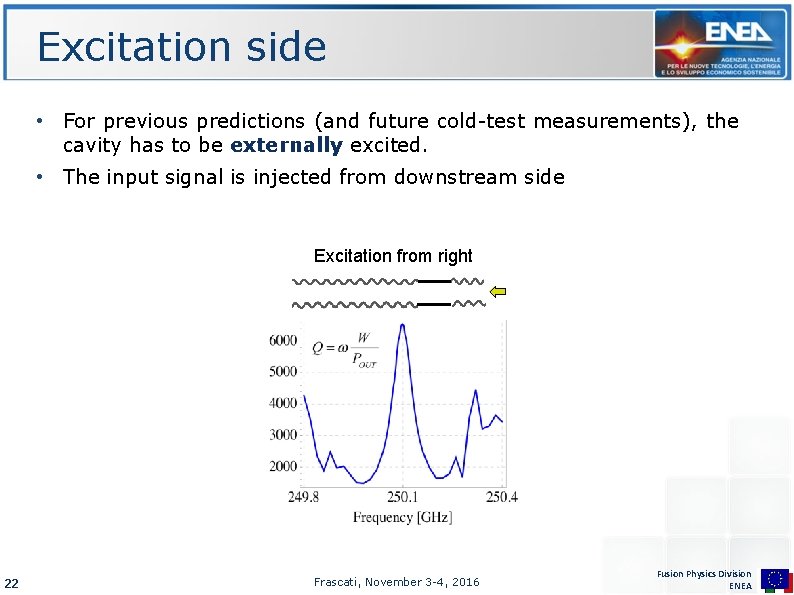
Excitation side • For previous predictions (and future cold-test measurements), the cavity has to be externally excited. • The input signal is injected from downstream side Excitation from right 22 Frascati, November 3 -4, 2016 Fusion Physics Division ENEA
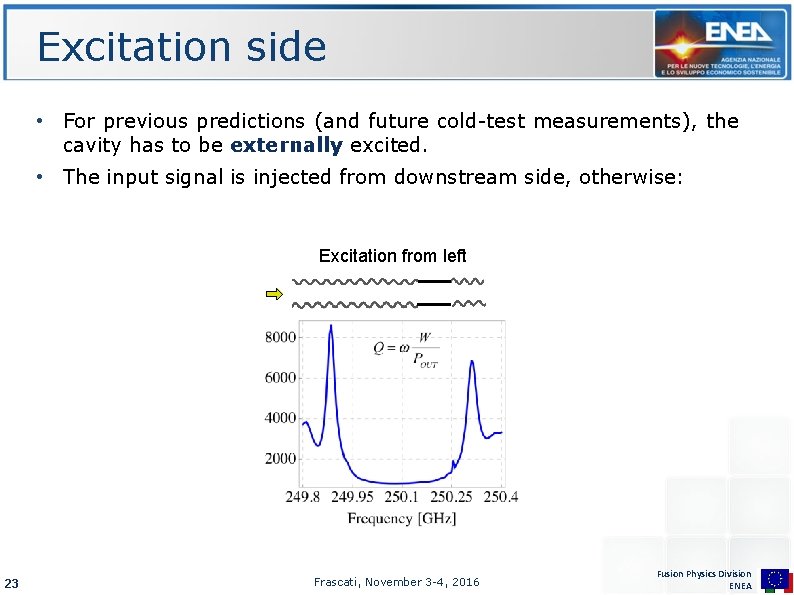
Excitation side • For previous predictions (and future cold-test measurements), the cavity has to be externally excited. • The input signal is injected from downstream side, otherwise: Excitation from left 23 Frascati, November 3 -4, 2016 Fusion Physics Division ENEA
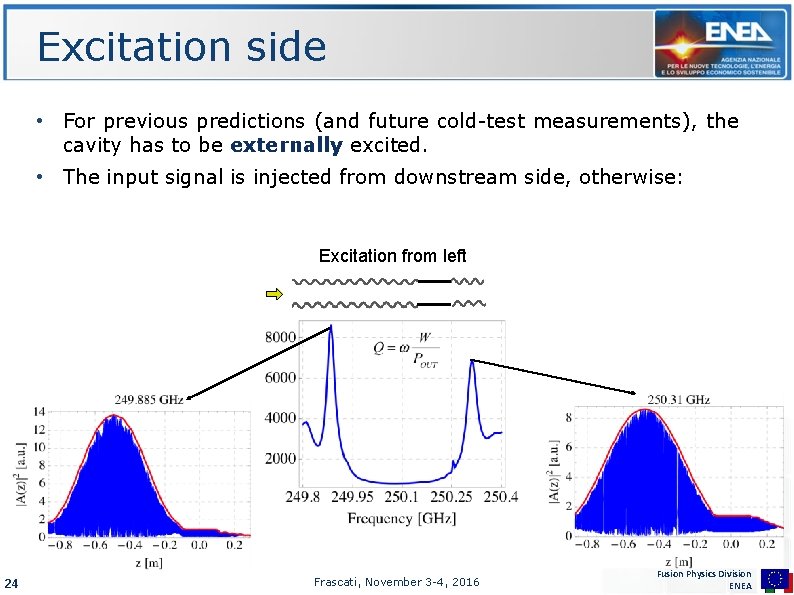
Excitation side • For previous predictions (and future cold-test measurements), the cavity has to be externally excited. • The input signal is injected from downstream side, otherwise: Excitation from left 24 Frascati, November 3 -4, 2016 Fusion Physics Division ENEA
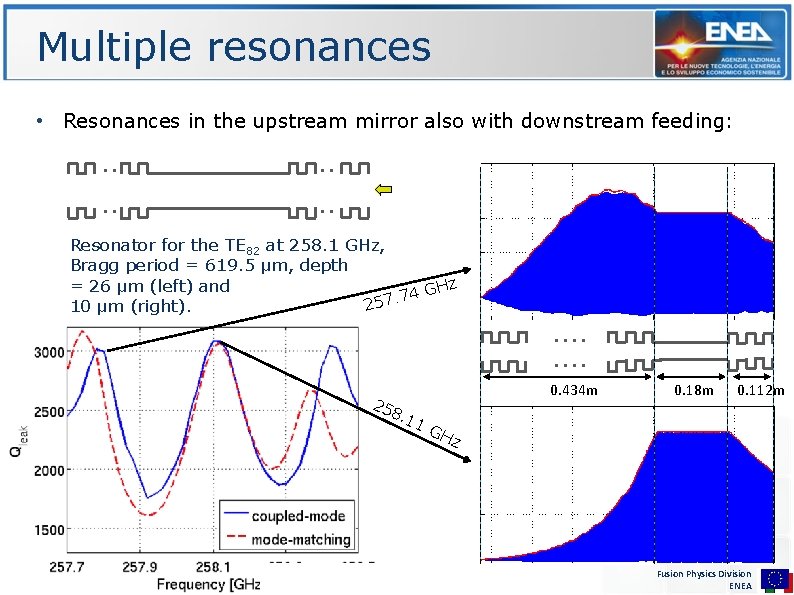
Multiple resonances • Resonances in the upstream mirror also with downstream feeding: Resonator for the TE 82 at 258. 1 GHz, Bragg period = 619. 5 μm, depth = 26 μm (left) and GHz 4 7. 257 10 μm (right). 25 0. 434 m 0. 18 m 0. 112 m 8. 1 25 1 G Hz Frascati, November 3 -4, 2016 Fusion Physics Division ENEA
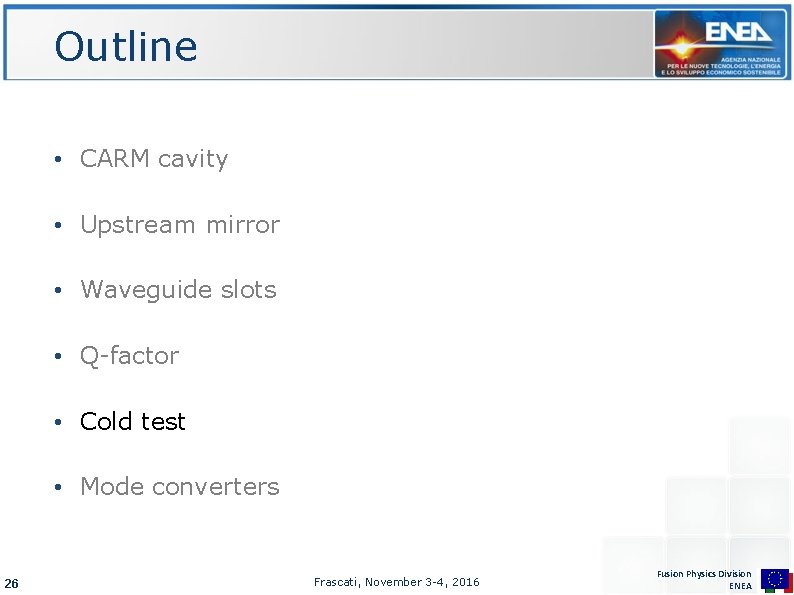
Outline • CARM cavity • Upstream mirror • Waveguide slots • Q-factor • Cold test • Mode converters 26 Frascati, November 3 -4, 2016 Fusion Physics Division ENEA
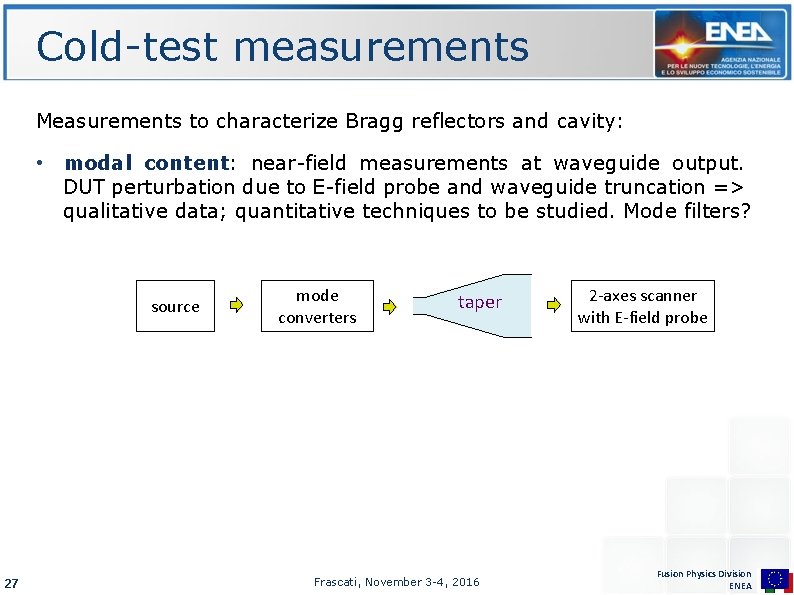
Cold-test measurements Measurements to characterize Bragg reflectors and cavity: • modal content: near-field measurements at waveguide output. DUT perturbation due to E-field probe and waveguide truncation => qualitative data; quantitative techniques to be studied. Mode filters? source 27 mode converters taper Frascati, November 3 -4, 2016 2 -axes scanner with E-field probe Fusion Physics Division ENEA
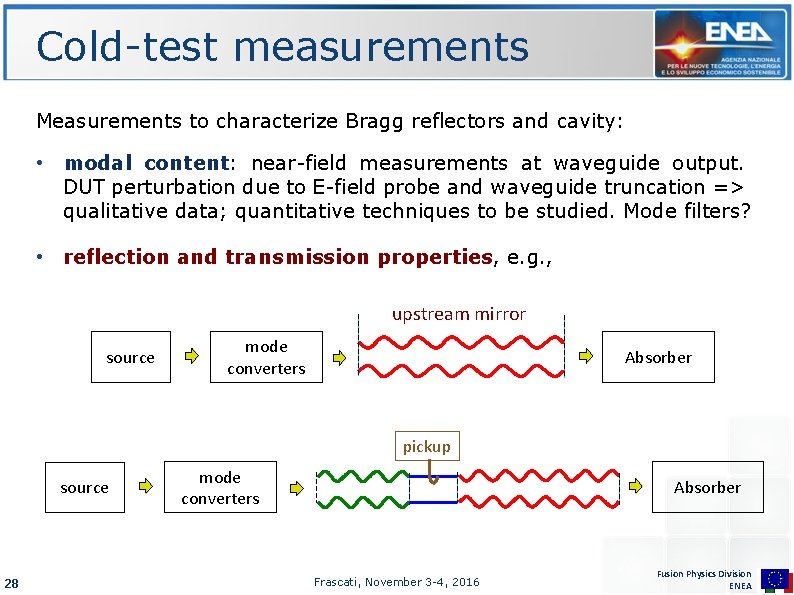
Cold-test measurements Measurements to characterize Bragg reflectors and cavity: • modal content: near-field measurements at waveguide output. DUT perturbation due to E-field probe and waveguide truncation => qualitative data; quantitative techniques to be studied. Mode filters? • reflection and transmission properties, e. g. , upstream mirror source mode converters Absorber pickup source 28 mode converters Absorber Frascati, November 3 -4, 2016 Fusion Physics Division ENEA
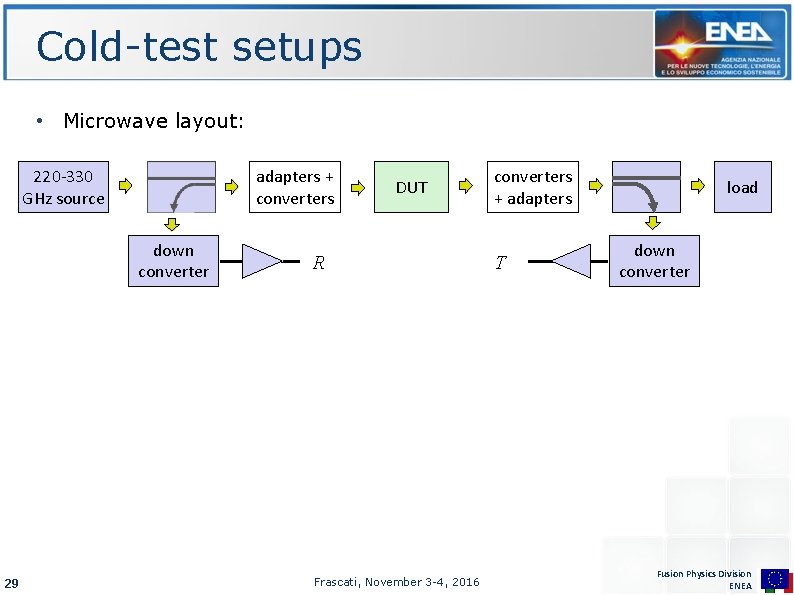
Cold-test setups • Microwave layout: adapters + converters 220 -330 GHz source down converter 29 DUT R Frascati, November 3 -4, 2016 converters + adapters T load down converter Fusion Physics Division ENEA
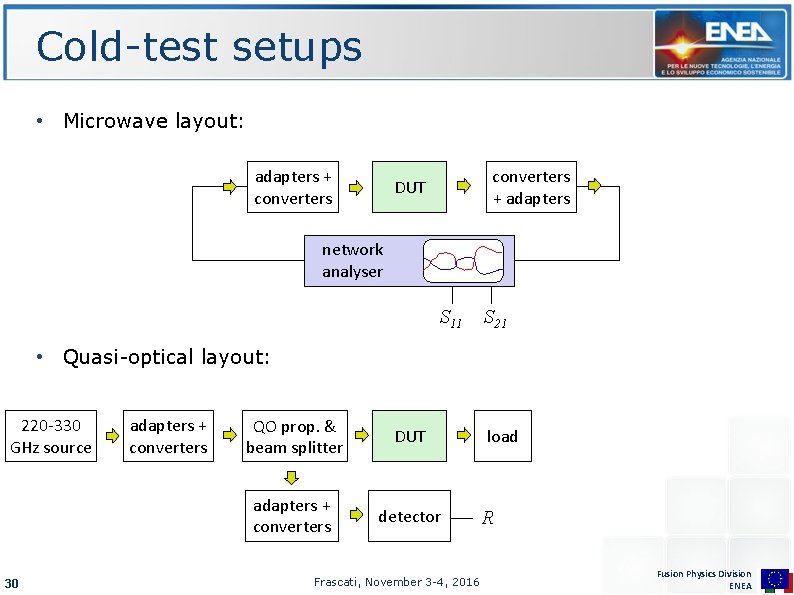
Cold-test setups • Microwave layout: adapters + converters + adapters DUT network analyser S 11 S 21 • Quasi-optical layout: 220 -330 GHz source 30 adapters + converters QO prop. & beam splitter DUT adapters + converters detector Frascati, November 3 -4, 2016 load R Fusion Physics Division ENEA
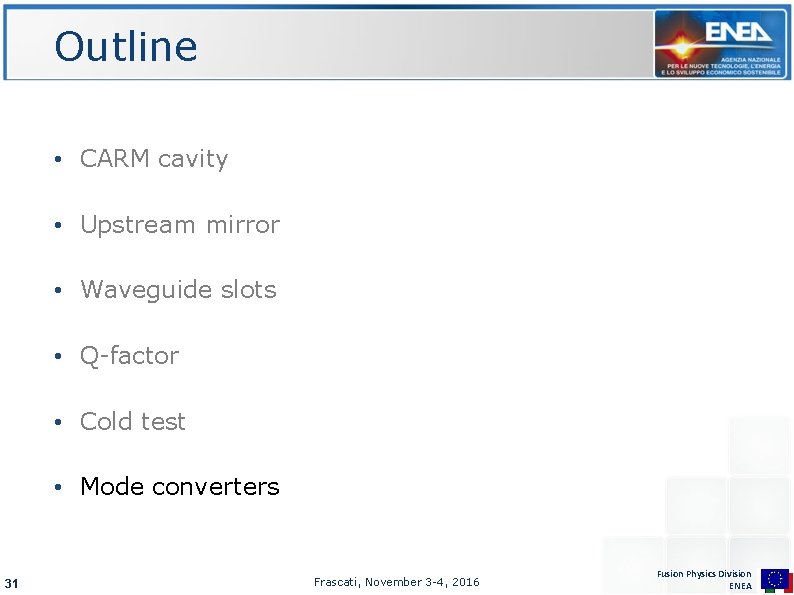
Outline • CARM cavity • Upstream mirror • Waveguide slots • Q-factor • Cold test • Mode converters 31 Frascati, November 3 -4, 2016 Fusion Physics Division ENEA
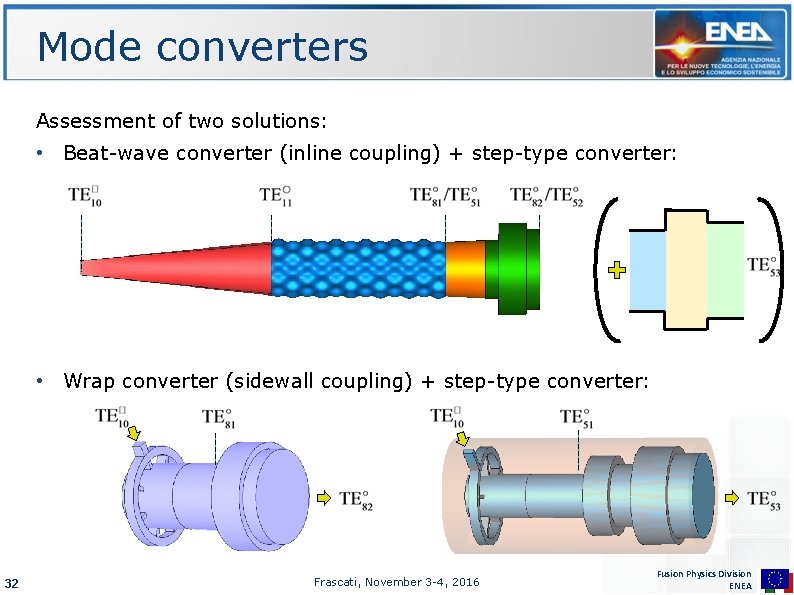
Mode converters Assessment of two solutions: • Beat-wave converter (inline coupling) + step-type converter: • Wrap converter (sidewall coupling) + step-type converter: 32 Frascati, November 3 -4, 2016 Fusion Physics Division ENEA
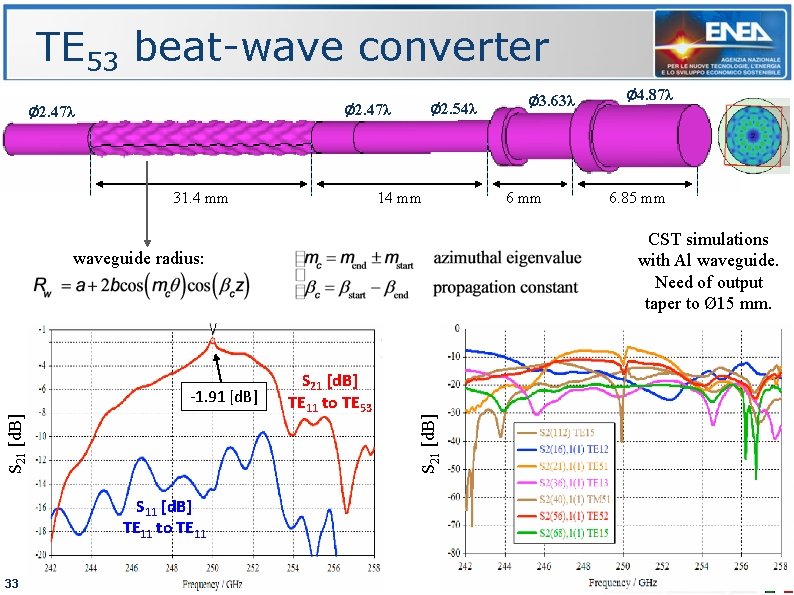
TE 53 beat-wave converter Ø 2. 54λ Ø 2. 47λ 31. 4 mm 14 mm 6. 85 mm S 21 [d. B] TE 11 to TE 53 S 21 [d. B] 6 mm Ø 4. 87λ CST simulations with Al waveguide. Need of output taper to Ø 15 mm. waveguide radius: -1. 91 [d. B] Ø 3. 63λ S 11 [d. B] TE 11 to TE 11 33 Frascati, November 3 -4, 2016 Fusion Physics Division ENEA
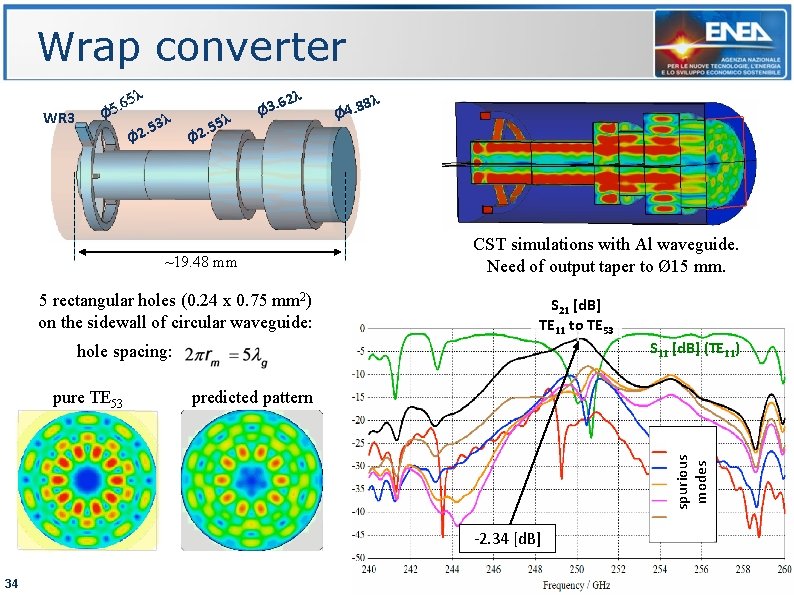
Wrap converter WR 3 5λ . 6 Ø 5 λ. 53 2 Ø 5λ . 5 Ø 2 62 Ø 3. λ ~19. 48 mm 88λ. 4 Ø CST simulations with Al waveguide. Need of output taper to Ø 15 mm. 5 rectangular holes (0. 24 x 0. 75 mm 2) on the sidewall of circular waveguide: S 21 [d. B] TE 11 to TE 53 S 11 [d. B] (TE 11) hole spacing: predicted pattern spurious modes pure TE 53 -2. 34 [d. B] 34 Frascati, November 3 -4, 2016 Fusion Physics Division ENEA
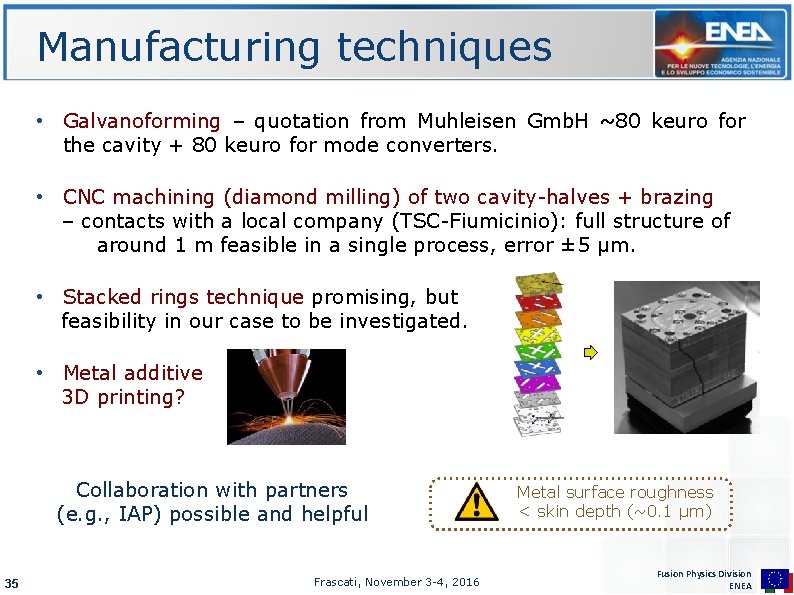
Manufacturing techniques • Galvanoforming – quotation from Muhleisen Gmb. H ~80 keuro for the cavity + 80 keuro for mode converters. • CNC machining (diamond milling) of two cavity-halves + brazing – contacts with a local company (TSC-Fiumicinio): full structure of around 1 m feasible in a single process, error ± 5 μm. • Stacked rings technique promising, but feasibility in our case to be investigated. • Metal additive 3 D printing? Collaboration with partners (e. g. , IAP) possible and helpful 35 Frascati, November 3 -4, 2016 Metal surface roughness < skin depth (~0. 1 μm) Fusion Physics Division ENEA
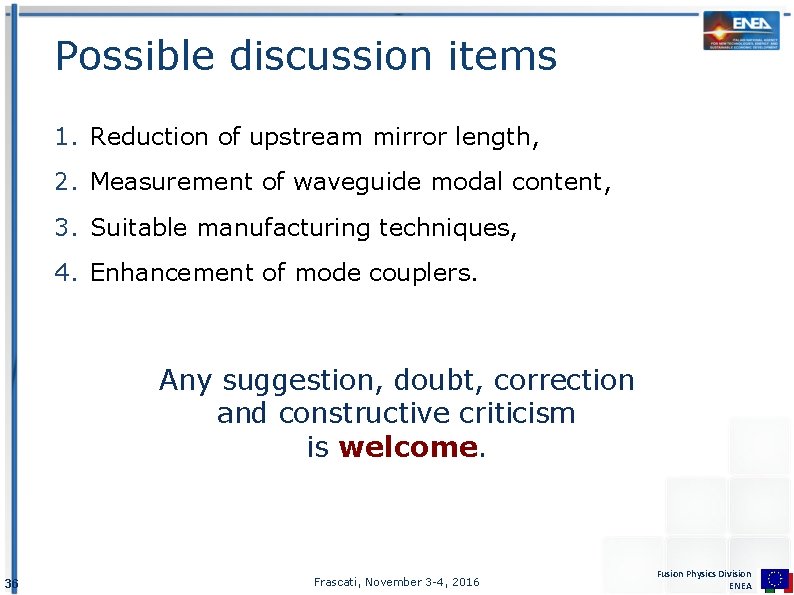
Possible discussion items 1. Reduction of upstream mirror length, 2. Measurement of waveguide modal content, 3. Suitable manufacturing techniques, 4. Enhancement of mode couplers. Any suggestion, doubt, correction and constructive criticism is welcome. 36 Frascati, November 3 -4, 2016 Fusion Physics Division ENEA
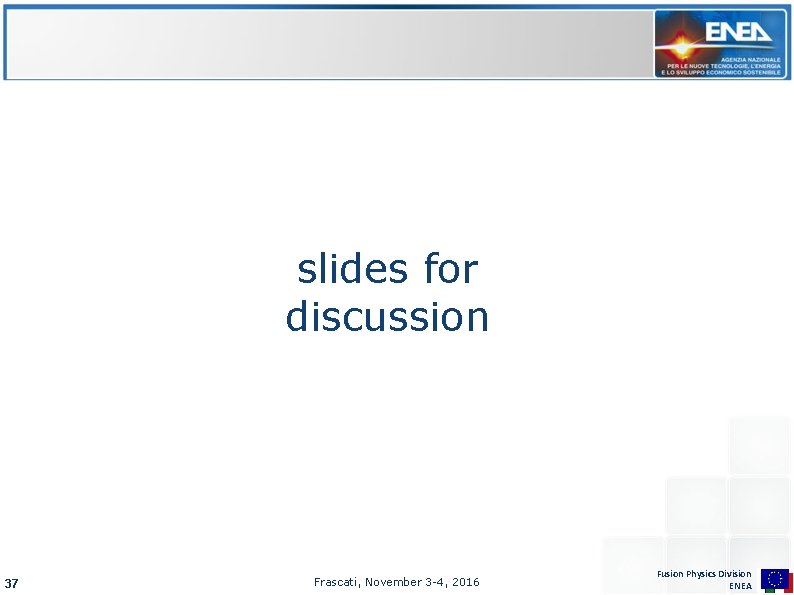
slides for discussion 37 Frascati, November 3 -4, 2016 Fusion Physics Division ENEA
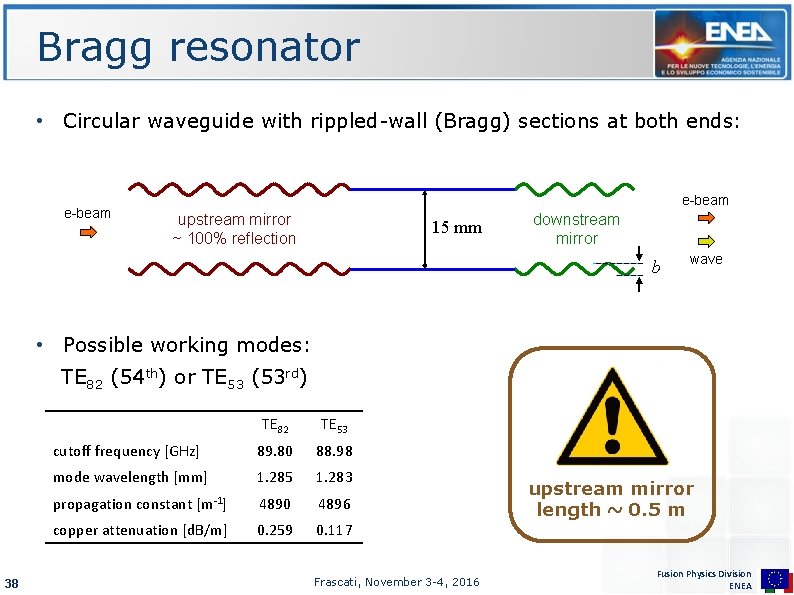
Bragg resonator • Circular waveguide with rippled-wall (Bragg) sections at both ends: e-beam upstream mirror ~ 100% reflection 15 mm downstream mirror b wave • Possible working modes: TE 82 (54 th) or TE 53 (53 rd) TE 82 TE 53 cutoff frequency [GHz] 89. 80 88. 98 mode wavelength [mm] 1. 285 1. 283 [m-1] 4890 4896 copper attenuation [d. B/m] 0. 259 0. 117 propagation constant 38 Frascati, November 3 -4, 2016 upstream mirror length ~ 0. 5 m Fusion Physics Division ENEA
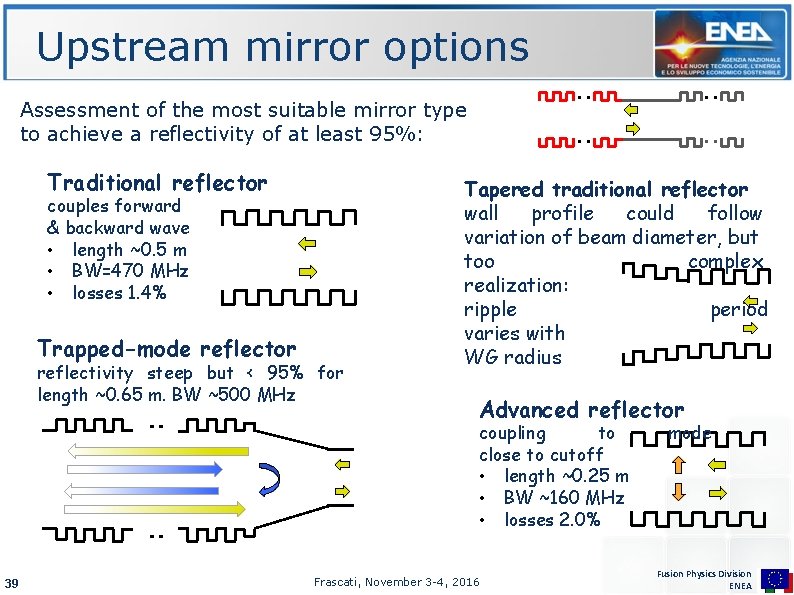
Upstream mirror options Assessment of the most suitable mirror type to achieve a reflectivity of at least 95%: Traditional reflector couples forward & backward wave • length ~0. 5 m • BW=470 MHz • losses 1. 4% Trapped-mode reflector reflectivity steep but < 95% for length ~0. 65 m. BW ~500 MHz Tapered traditional reflector wall profile could follow variation of beam diameter, but too complex realization: ripple period varies with WG radius Advanced reflector coupling to close to cutoff • length ~0. 25 m • BW ~160 MHz • losses 2. 0% 39 Frascati, November 3 -4, 2016 mode Fusion Physics Division ENEA
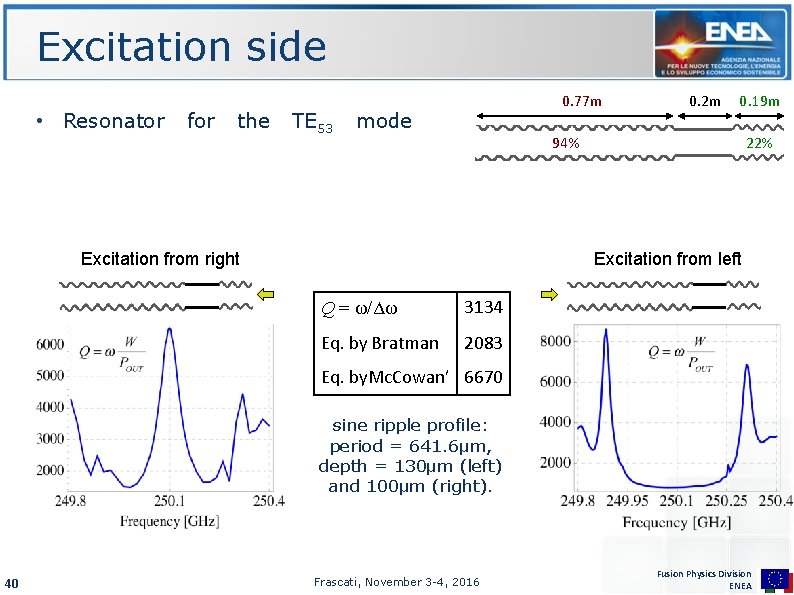
Excitation side • Resonator for the TE 53 0. 77 m mode 0. 2 m 0. 19 m 94% Excitation from right 22% Excitation from left Q = ω/Δω 3134 Eq. by Bratman 2083 Eq. by. Mc. Cowan’ 6670 sine ripple profile: period = 641. 6μm, depth = 130μm (left) and 100μm (right). 40 Frascati, November 3 -4, 2016 Fusion Physics Division ENEA
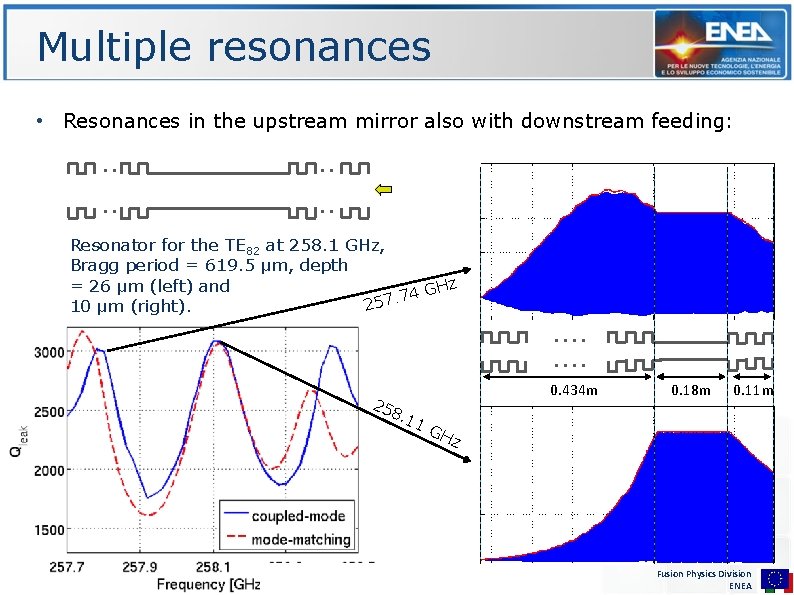
Multiple resonances • Resonances in the upstream mirror also with downstream feeding: Resonator for the TE 82 at 258. 1 GHz, Bragg period = 619. 5 μm, depth = 26 μm (left) and GHz 4 7. 257 10 μm (right). 25 0. 434 m 0. 18 m 0. 11 m 8. 1 41 1 G Hz Frascati, November 3 -4, 2016 Fusion Physics Division ENEA
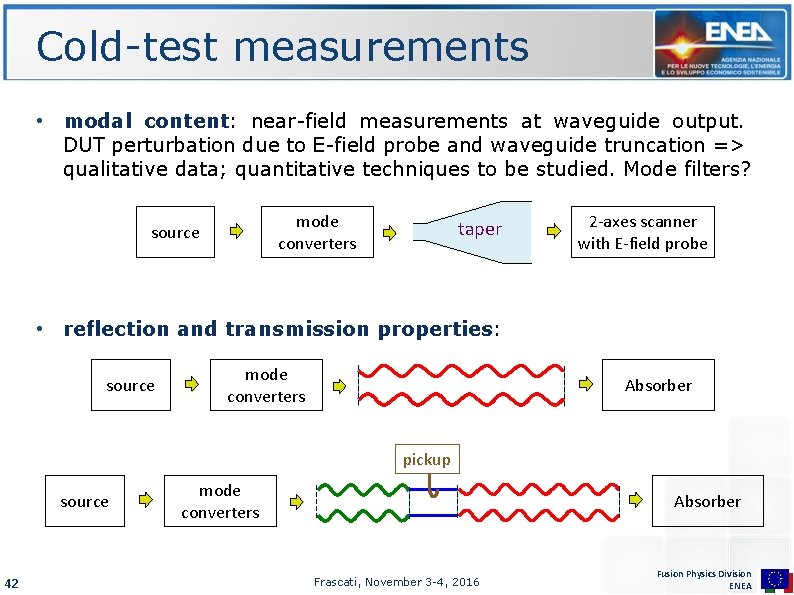
Cold-test measurements • modal content: near-field measurements at waveguide output. DUT perturbation due to E-field probe and waveguide truncation => qualitative data; quantitative techniques to be studied. Mode filters? mode converters source taper 2 -axes scanner with E-field probe • reflection and transmission properties: source mode converters Absorber pickup source 42 mode converters Absorber Frascati, November 3 -4, 2016 Fusion Physics Division ENEA
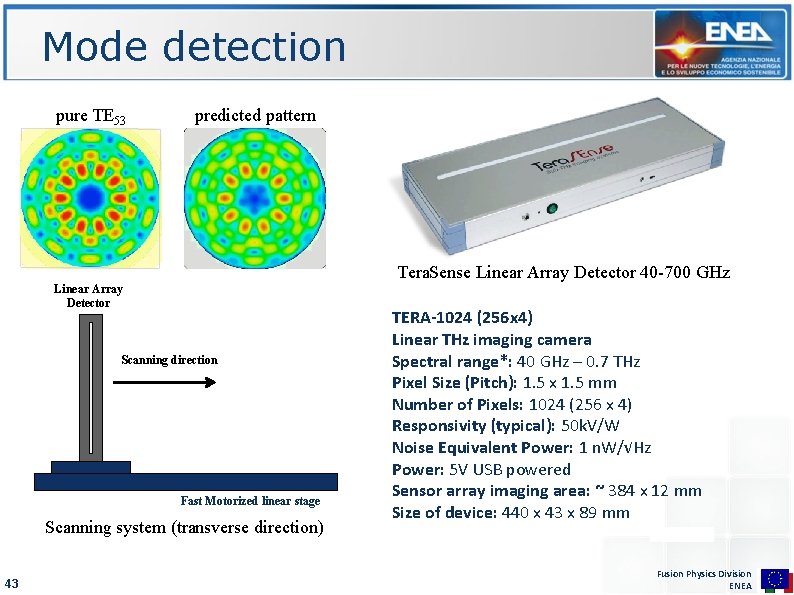
Mode detection pure TE 53 predicted pattern Tera. Sense Linear Array Detector 40 -700 GHz Linear Array Detector Scanning direction Fast Motorized linear stage Scanning system (transverse direction) 43 TERA-1024 (256 x 4) Linear THz imaging camera Spectral range*: 40 GHz – 0. 7 THz Pixel Size (Pitch): 1. 5 x 1. 5 mm Number of Pixels: 1024 (256 x 4) Responsivity (typical): 50 k. V/W Noise Equivalent Power: 1 n. W/√Hz Power: 5 V USB powered Sensor array imaging area: ~ 384 x 12 mm Size of device: 440 x 43 x 89 mm Frascati, November 3 -4, 2016 Fusion Physics Division ENEA
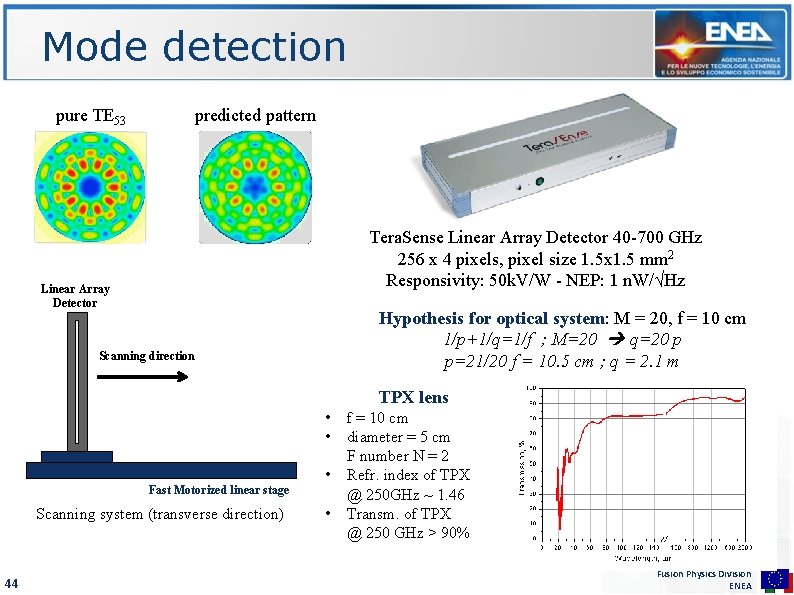
Mode detection pure TE 53 predicted pattern Tera. Sense Linear Array Detector 40 -700 GHz 256 x 4 pixels, pixel size 1. 5 x 1. 5 mm 2 Responsivity: 50 k. V/W - NEP: 1 n. W/√Hz Linear Array Detector Hypothesis for optical system: M = 20, f = 10 cm 1/p+1/q=1/f ; M=20 q=20 p p=21/20 f = 10. 5 cm ; q = 2. 1 m Scanning direction TPX lens • • Fast Motorized linear stage Scanning system (transverse direction) 44 • • f = 10 cm diameter = 5 cm F number N = 2 Refr. index of TPX @ 250 GHz ~ 1. 46 Transm. of TPX @ 250 GHz > 90% Frascati, November 3 -4, 2016 Fusion Physics Division ENEA
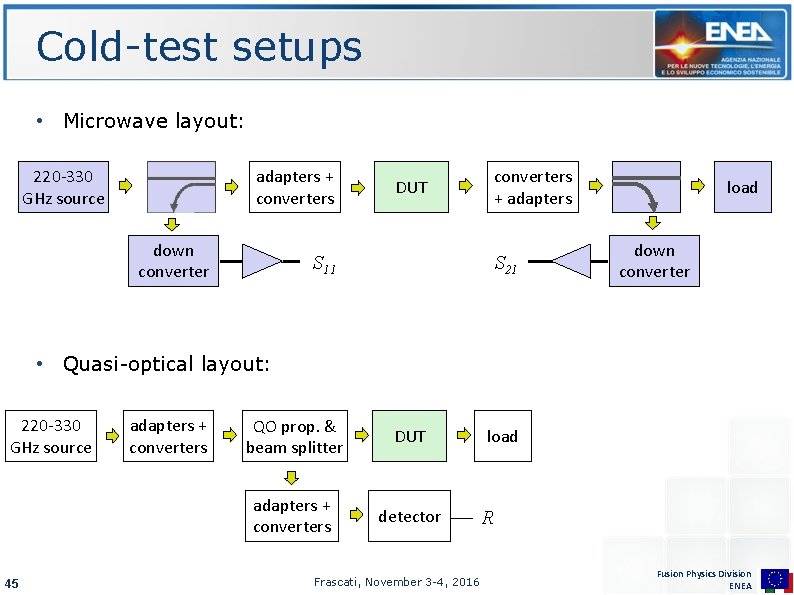
Cold-test setups • Microwave layout: adapters + converters 220 -330 GHz source down converter DUT converters + adapters S 11 S 21 load down converter • Quasi-optical layout: 220 -330 GHz source 45 adapters + converters QO prop. & beam splitter DUT adapters + converters detector Frascati, November 3 -4, 2016 load R Fusion Physics Division ENEA
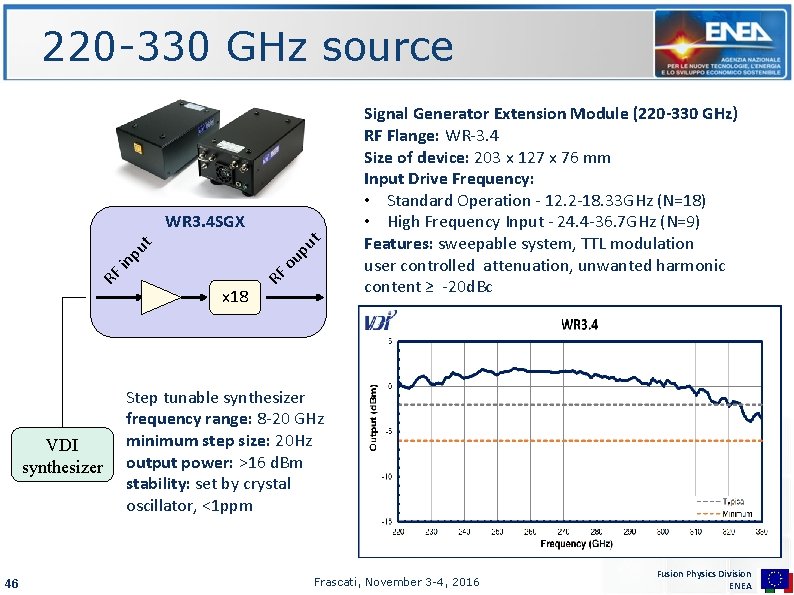
220 -330 GHz source VDI synthesizer 46 x 18 RF RF in ou pu pu t t WR 3. 4 SGX Signal Generator Extension Module (220 -330 GHz) RF Flange: WR-3. 4 Size of device: 203 x 127 x 76 mm Input Drive Frequency: • Standard Operation - 12. 2 -18. 33 GHz (N=18) • High Frequency Input - 24. 4 -36. 7 GHz (N=9) Features: sweepable system, TTL modulation user controlled attenuation, unwanted harmonic content ≥ -20 d. Bc Step tunable synthesizer frequency range: 8 -20 GHz minimum step size: 20 Hz output power: >16 d. Bm stability: set by crystal oscillator, <1 ppm Frascati, November 3 -4, 2016 Fusion Physics Division ENEA
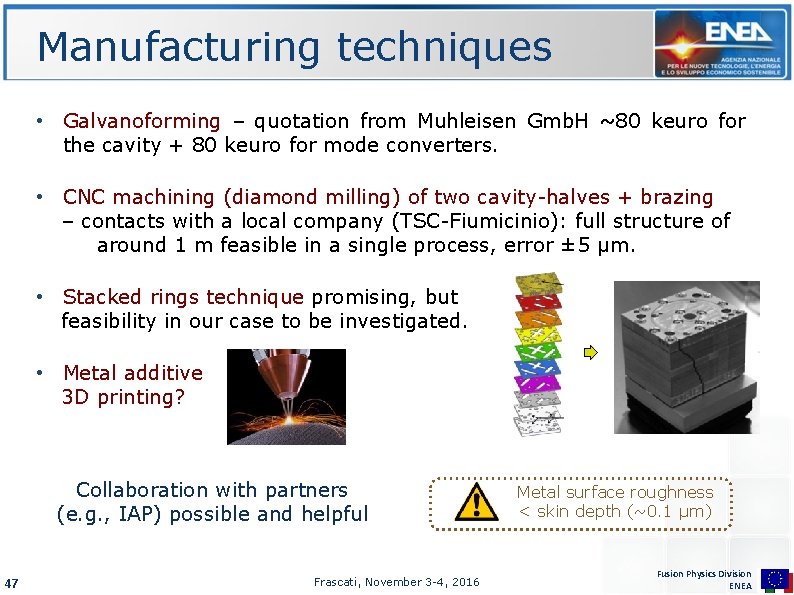
Manufacturing techniques • Galvanoforming – quotation from Muhleisen Gmb. H ~80 keuro for the cavity + 80 keuro for mode converters. • CNC machining (diamond milling) of two cavity-halves + brazing – contacts with a local company (TSC-Fiumicinio): full structure of around 1 m feasible in a single process, error ± 5 μm. • Stacked rings technique promising, but feasibility in our case to be investigated. • Metal additive 3 D printing? Collaboration with partners (e. g. , IAP) possible and helpful 47 Frascati, November 3 -4, 2016 Metal surface roughness < skin depth (~0. 1 μm) Fusion Physics Division ENEA
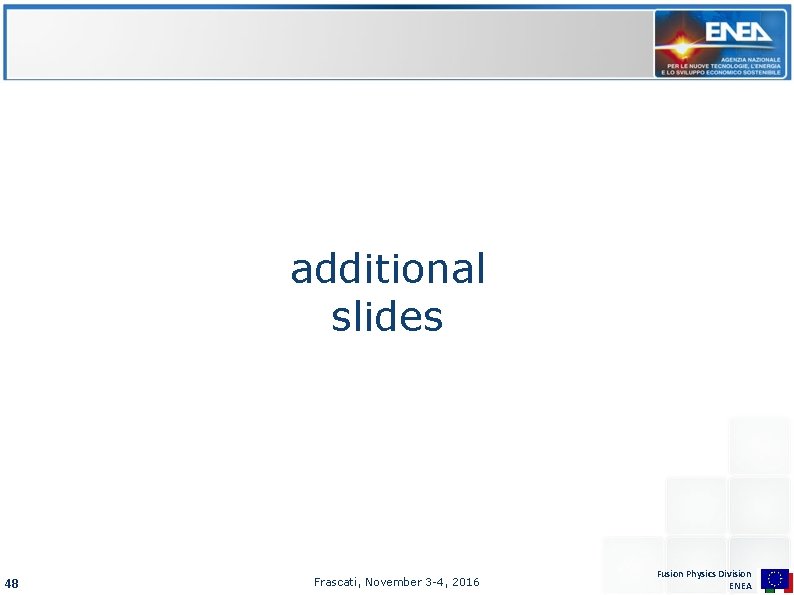
additional slides 48 Frascati, November 3 -4, 2016 Fusion Physics Division ENEA
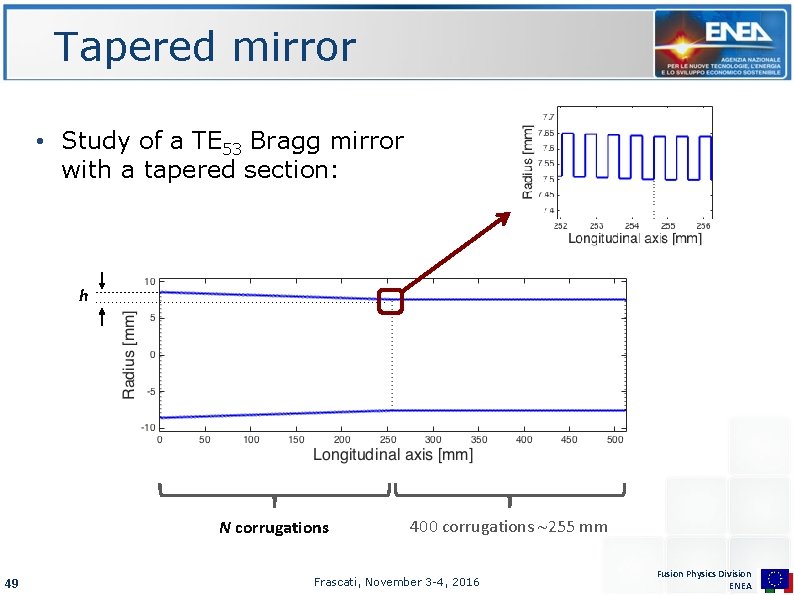
Tapered mirror • Study of a TE 53 Bragg mirror with a tapered section: h N corrugations 49 400 corrugations ~255 mm Frascati, November 3 -4, 2016 Fusion Physics Division ENEA
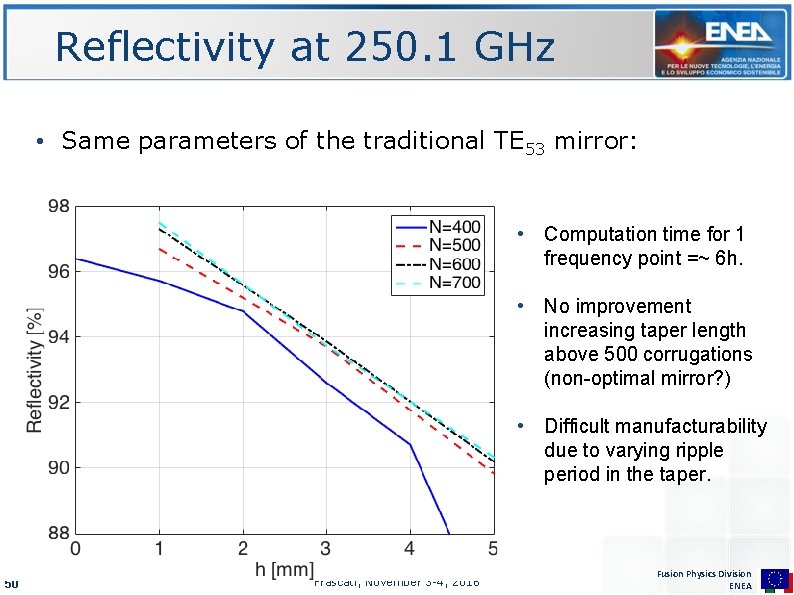
Reflectivity at 250. 1 GHz • Same parameters of the traditional TE 53 mirror: • Computation time for 1 frequency point =~ 6 h. • No improvement increasing taper length above 500 corrugations (non-optimal mirror? ) • Difficult manufacturability due to varying ripple period in the taper. 50 Frascati, November 3 -4, 2016 Fusion Physics Division ENEA
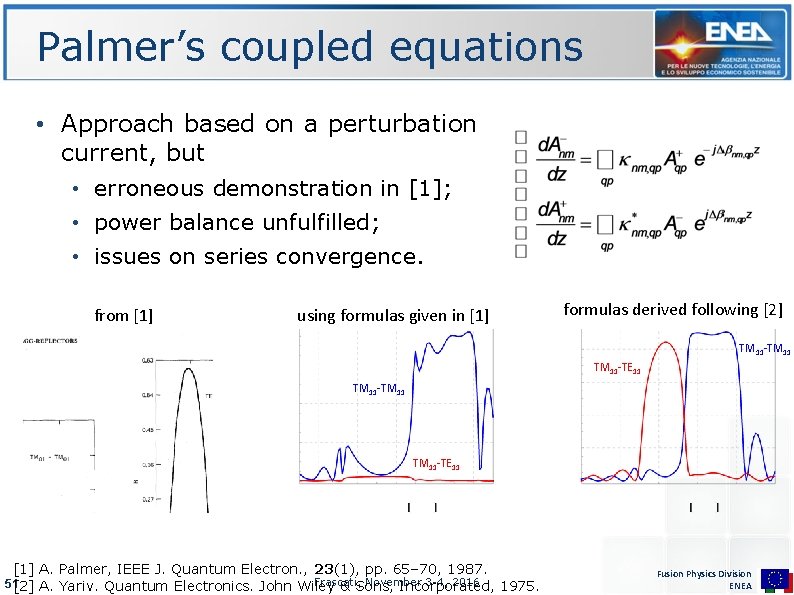
Palmer’s coupled equations • Approach based on a perturbation current, but • erroneous demonstration in [1]; • power balance unfulfilled; • issues on series convergence. from [1] (a) using formulas given in [1] (b) formulas derived following [2] (c) TM 11 -TM 11 -TE 11 [1] A. Palmer, IEEE J. Quantum Electron. , 23(1), pp. 65– 70, 1987. Frascati, November 3 -4, 2016 51[2] A. Yariv. Quantum Electronics. John Wiley & Sons, Incorporated, 1975. Fusion Physics Division ENEA
![Solymars coupled equations Widespread approach 1 2 used by Mc Cowan Thumm Solymar’s coupled equations • Widespread approach [1, 2], used by Mc. Cowan, Thumm, …](https://slidetodoc.com/presentation_image_h2/9c82b675c66be87ef7f1b4dc394a2855/image-52.jpg)
Solymar’s coupled equations • Widespread approach [1, 2], used by Mc. Cowan, Thumm, … • The coupled-mode equations were derived, obtaining similar coupling constants to the ones given in literature: Λ 2 b a 0 A+ z A- β = phase constant α = attenuation coefficient g 1 = 1 st Fourier coefficient of the k 0 = ω/c [ripple profile u’nm, unm = m-th zero of J’n and Jn Gip = coupling constants [1] L. Solymar, IRE Trans. on Microwave Theory and Techn. , 7(3), pp. 379– 383, 1959. Frascati, November 3 -4, 2016 52[2] C. K. Chong et al. , IEEE Trans. on Plasma Science, 20(3), pp. 393– 402, 1992. Fusion Physics Division ENEA
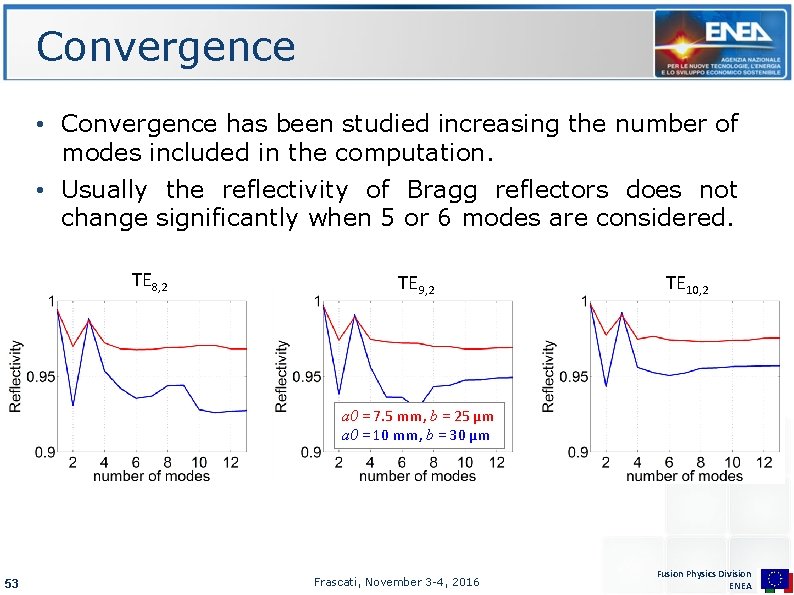
Convergence • Convergence has been studied increasing the number of modes included in the computation. • Usually the reflectivity of Bragg reflectors does not change significantly when 5 or 6 modes are considered. TE 8, 2 TE 9, 2 TE 10, 2 a 0 = 7. 5 mm, b = 25 μm a 0 = 10 mm, b = 30 μm 53 Frascati, November 3 -4, 2016 Fusion Physics Division ENEA
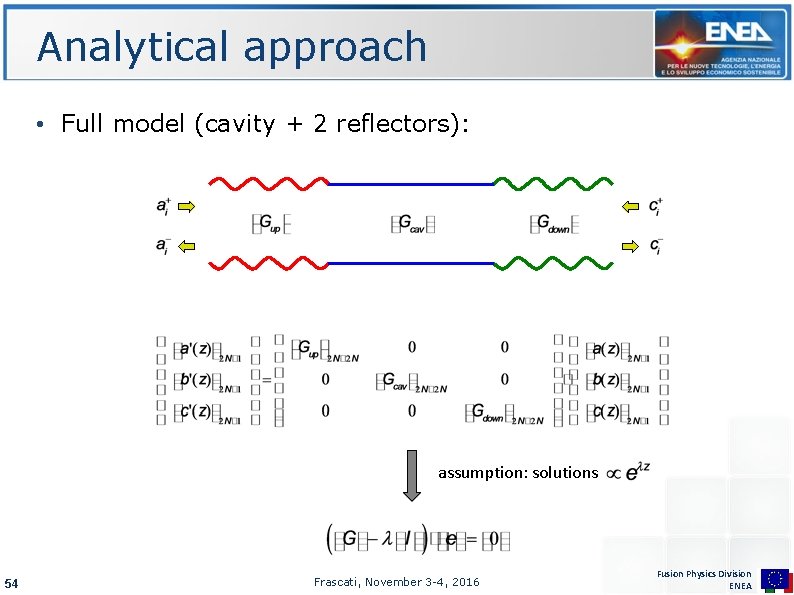
Analytical approach • Full model (cavity + 2 reflectors): assumption: solutions 54 Frascati, November 3 -4, 2016 Fusion Physics Division ENEA
Body cavities labeled
Imaginary line through body
Abdominopelvic cavity regions
Abdominal cavity
What is epiploic foramen
Lnf mensa
Doc phone frascati
Silvio frascati
Bpm frascati
Gigamail enea
Codice ricevuta enea esempio
Enea lungotevere thaon di revel 76
Viaggio di enea mappa
Enea
Espa enea
Andrea beatrice chiara davide enea
Nelissen ingenieursbureau
Rangkaian resonator
Closed-pipe resonator
Split cylinder resonator
Vacuum cleaner motor project
Open pipe resonator example
Harmonica resonator
Complementary split ring resonator
Lumped element resonator
Krasner and rankow law of access opening
Pharynx to esophagus
Hollow ground chamfer
Z puller pin design
Landmarks of the face and oral cavity chapter 10
Pulp protection liners and bases
Class iii preparation
Cell phase
Black's classification of cavities
Equazione di boltzmann
Nine regions of the abdominopelvic cavity
Muscularis externa
Sibsons fascia
Finesse of a cavity
Die cavity tablet
Sponge gastrovascular cavity
Stomach physiology
Pore bearers cannot move
Isthmus of tooth preparation
Incisive foramen contents
Palatopharyngeal arch
Venous drainage of the nose
Dr mujahid nazal
Sphenoid bone anatomy
Nasal cavity communications
Finesse of a cavity
Pig thoracic cavity diagram
Retention coves
Cavity ring down
Cervical esophagus