CHAPTER 5 GASES 5 1 PRESSURE Properties Gases
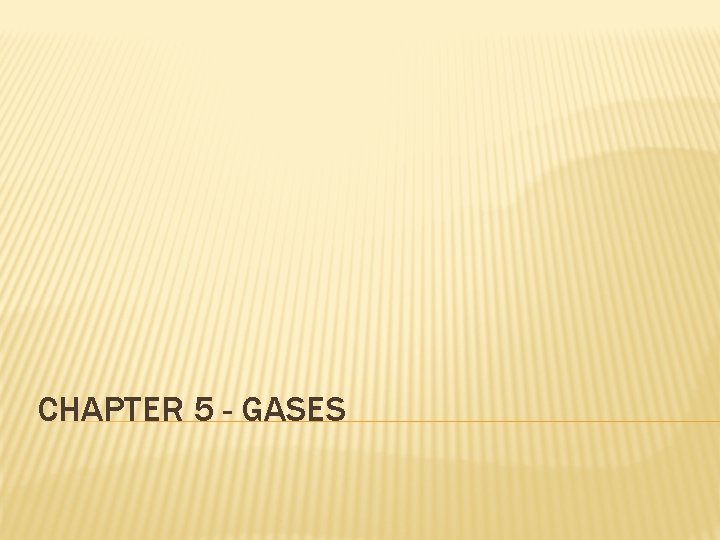
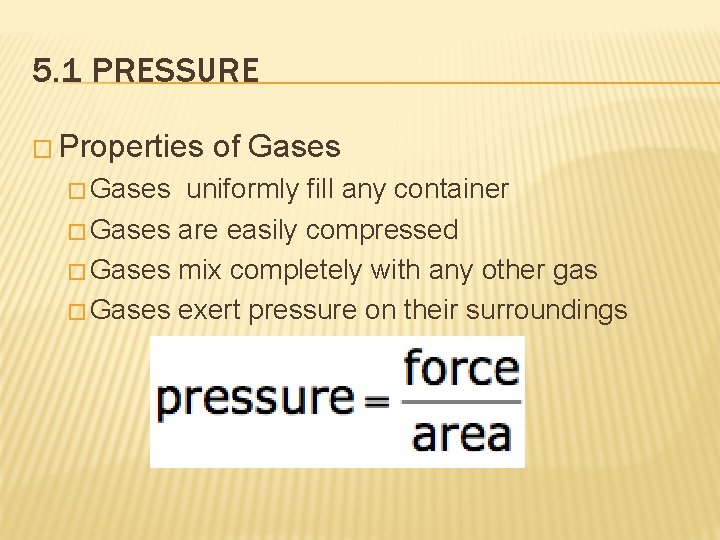
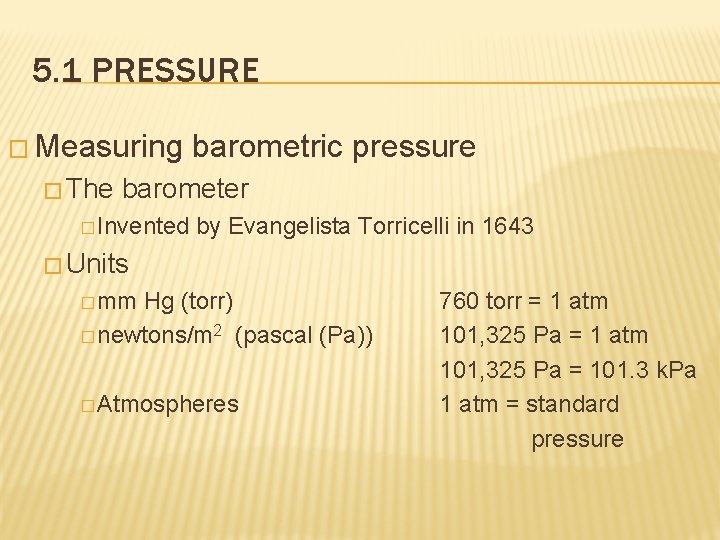
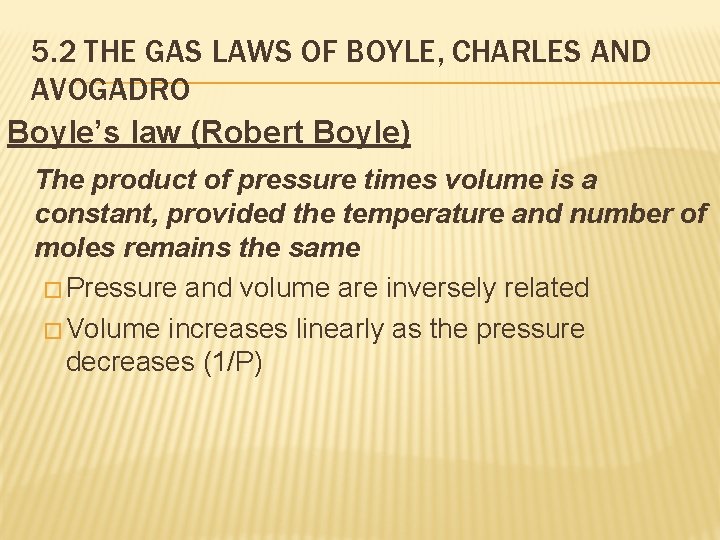
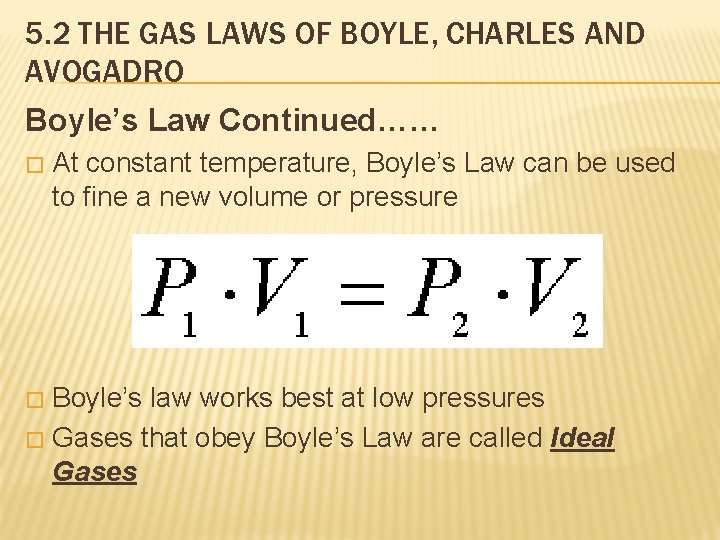
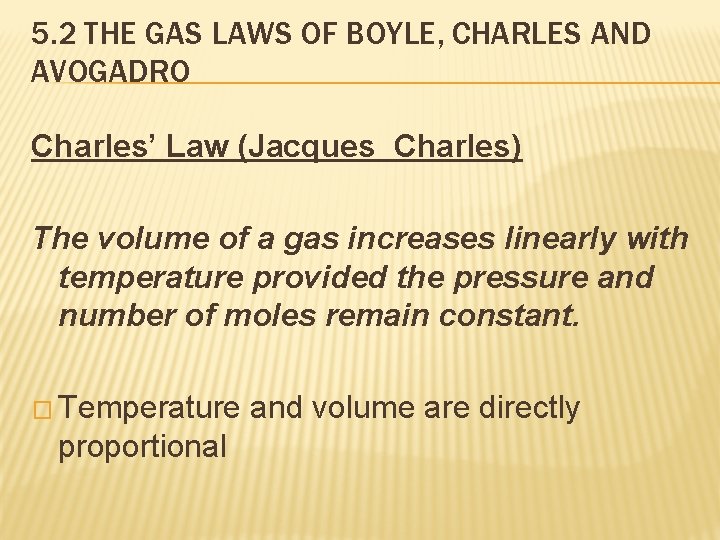
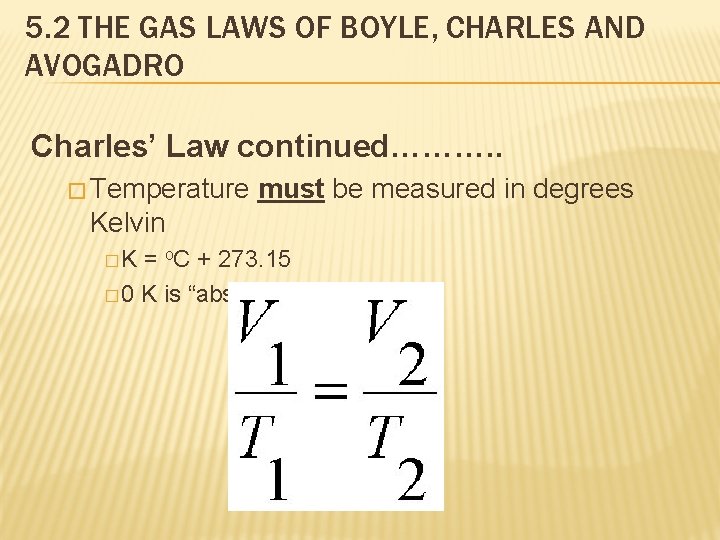
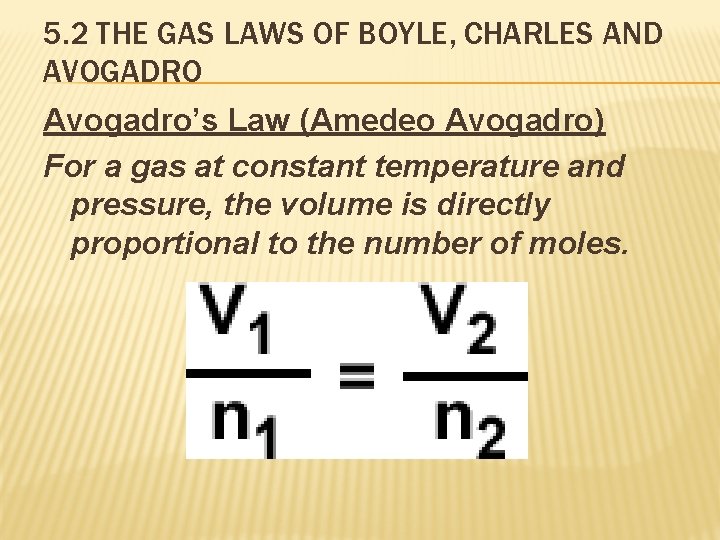
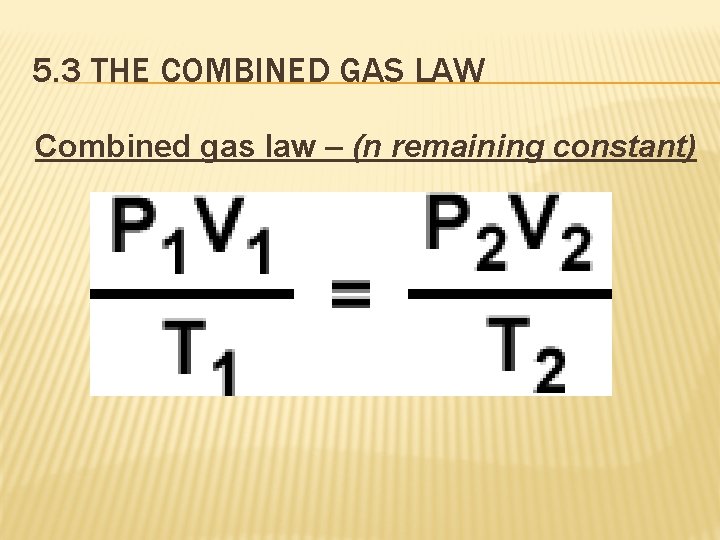
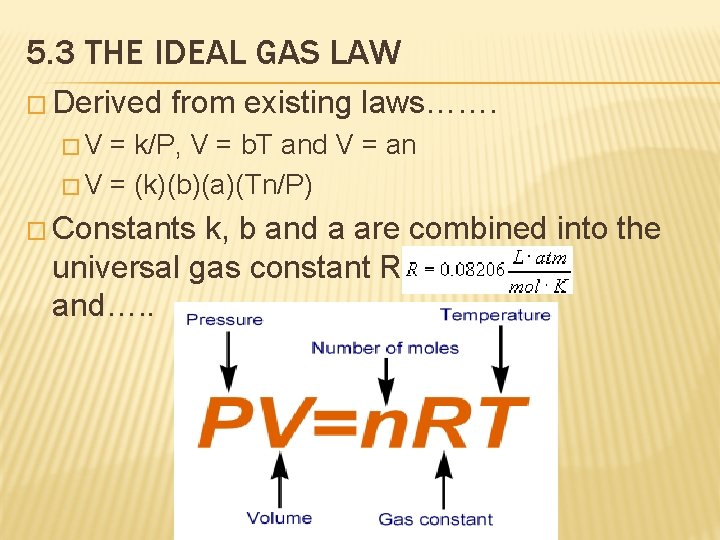
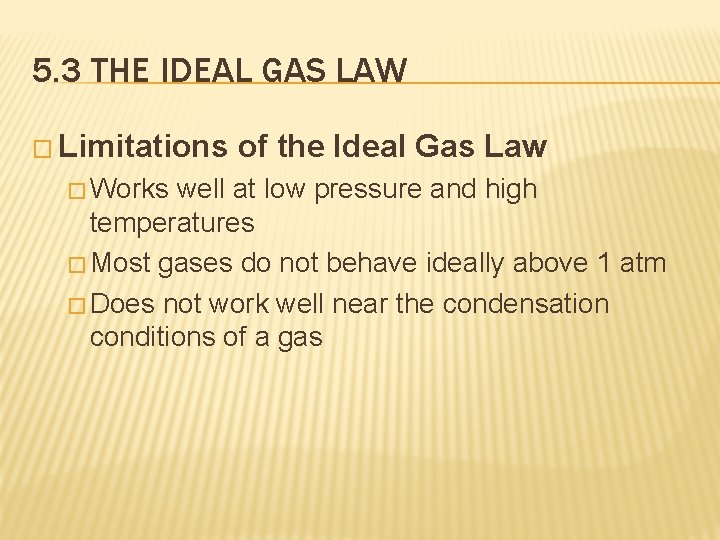
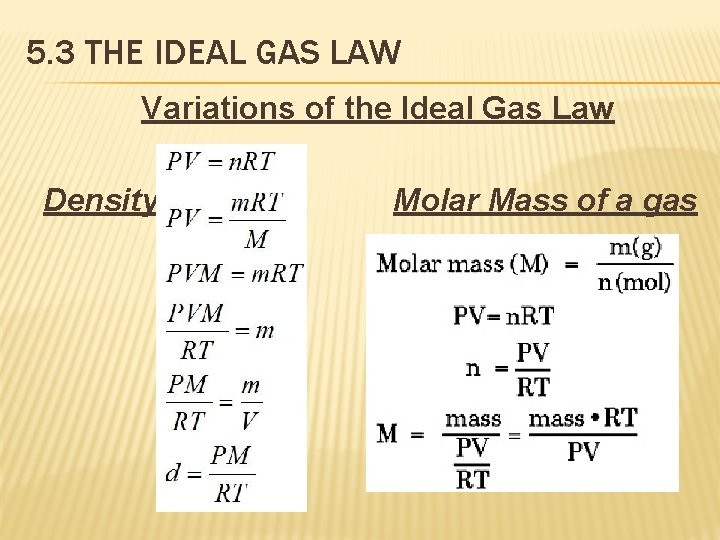
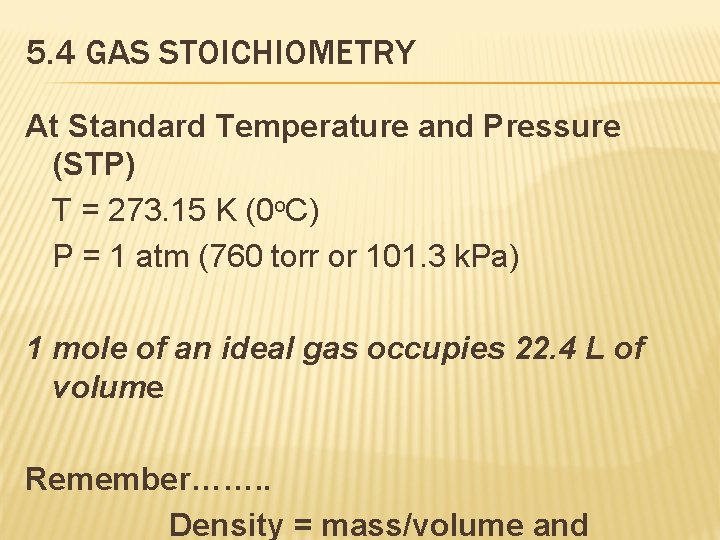
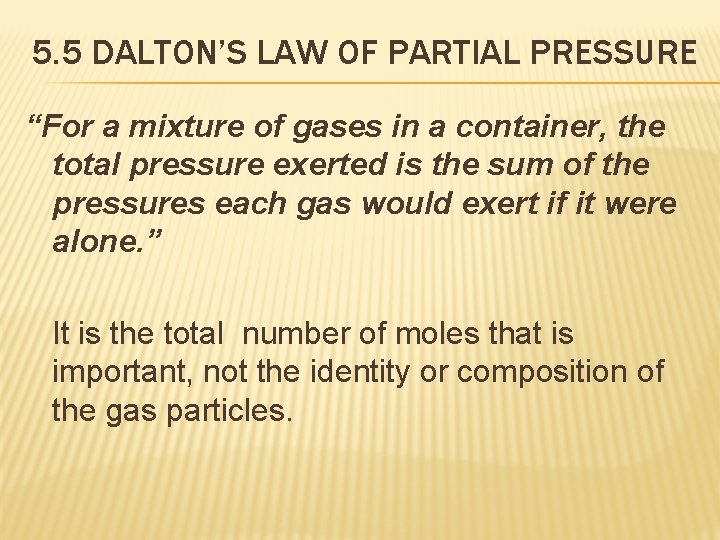
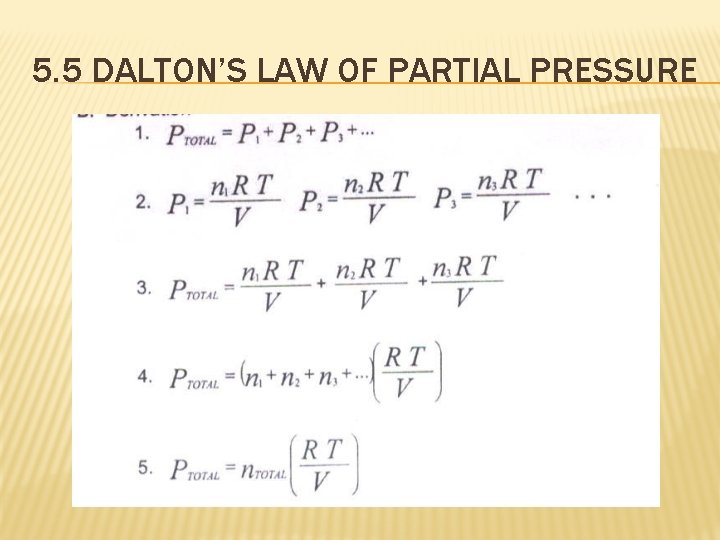
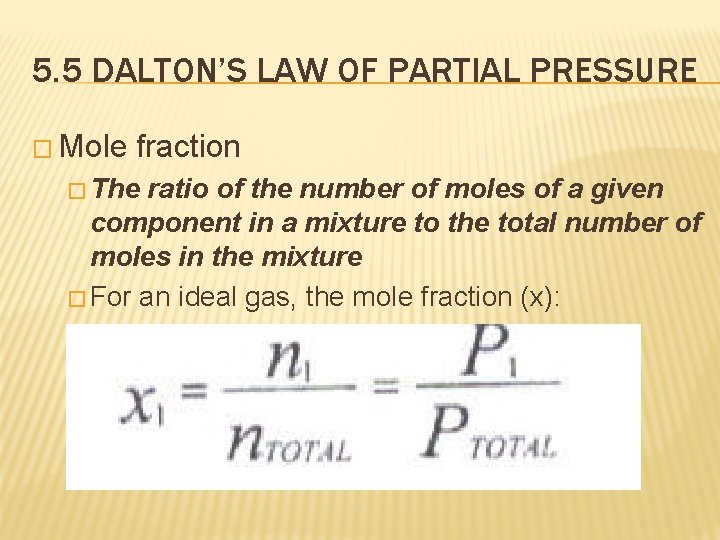
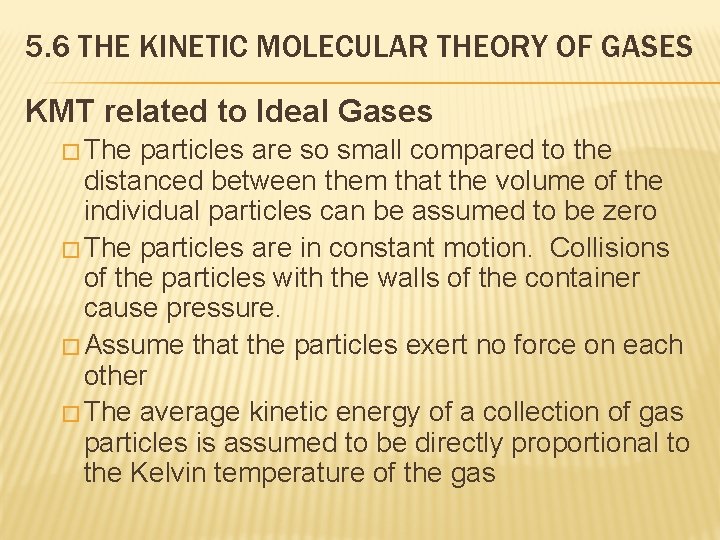
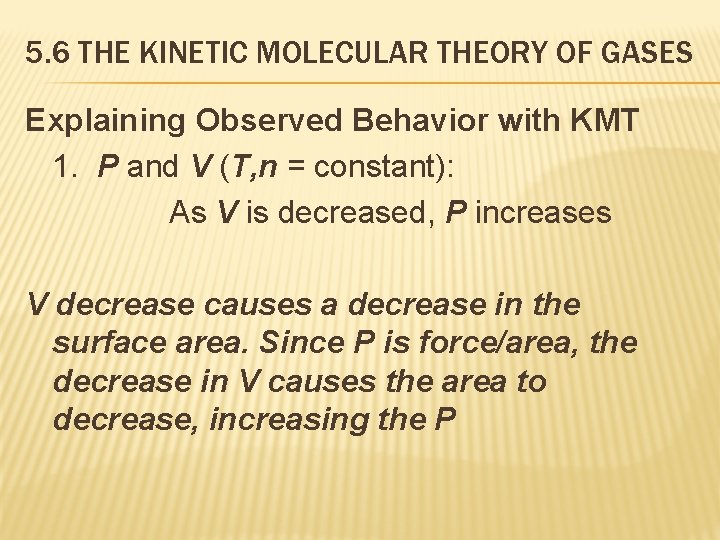
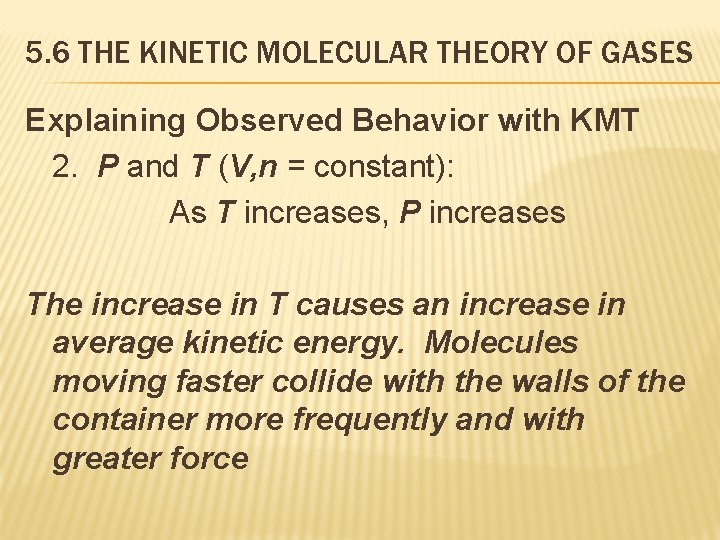
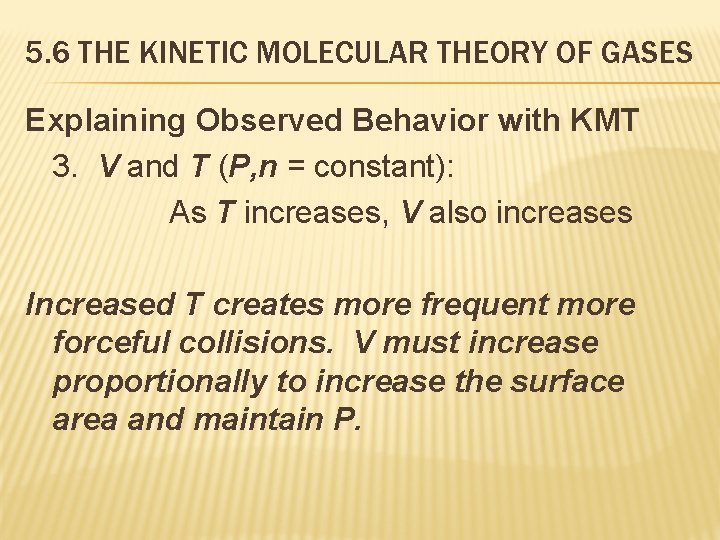
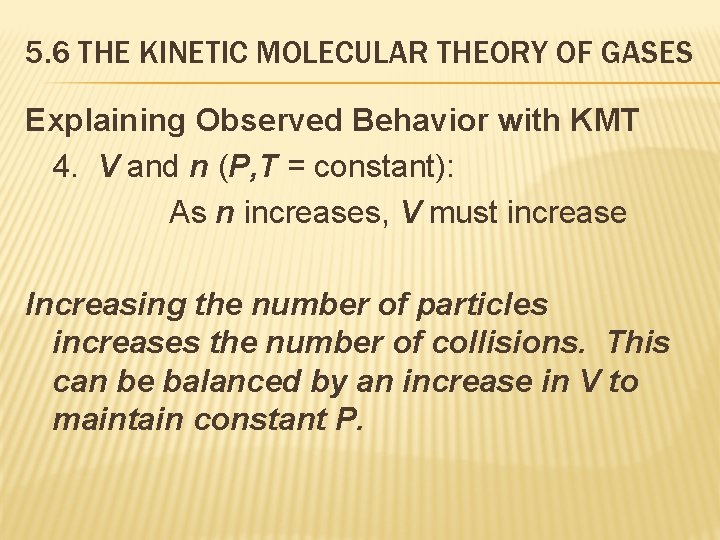
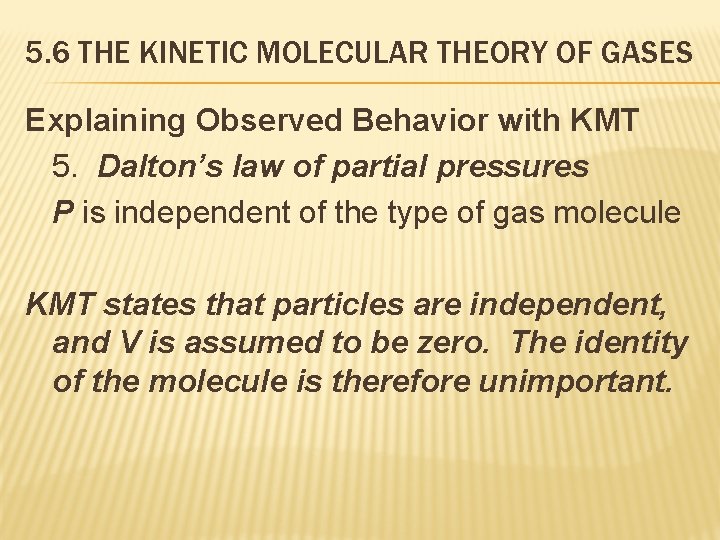
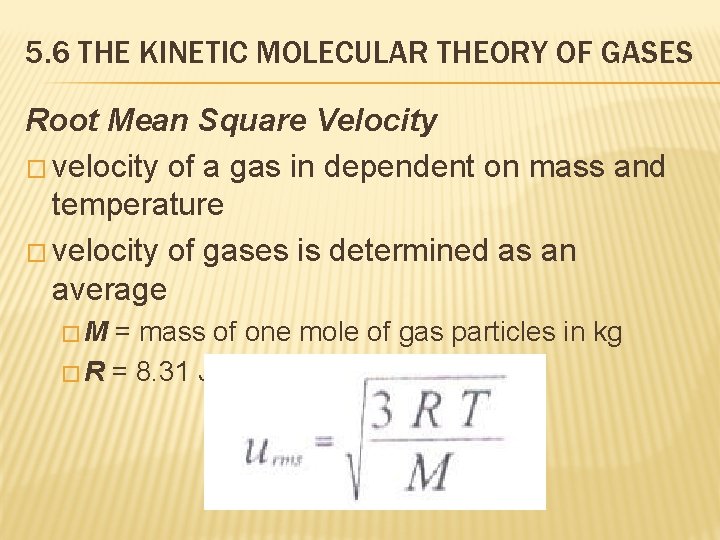
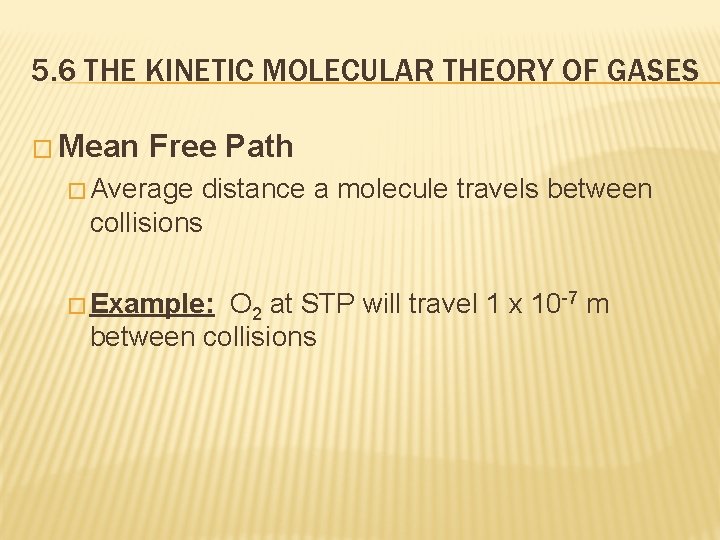
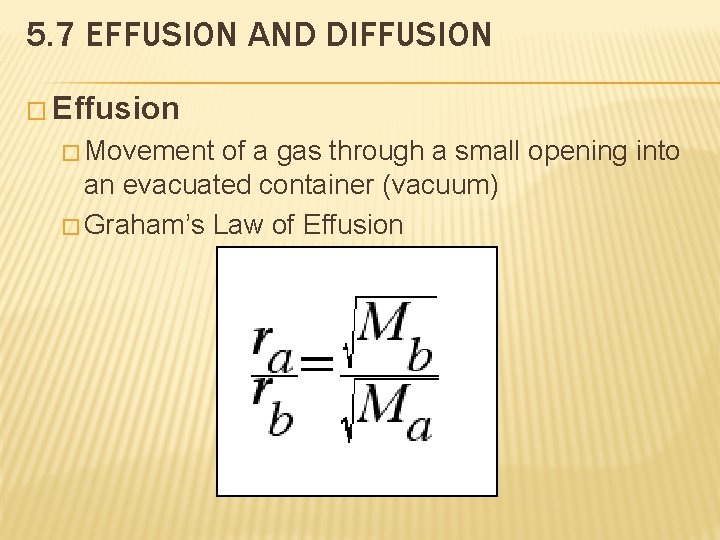
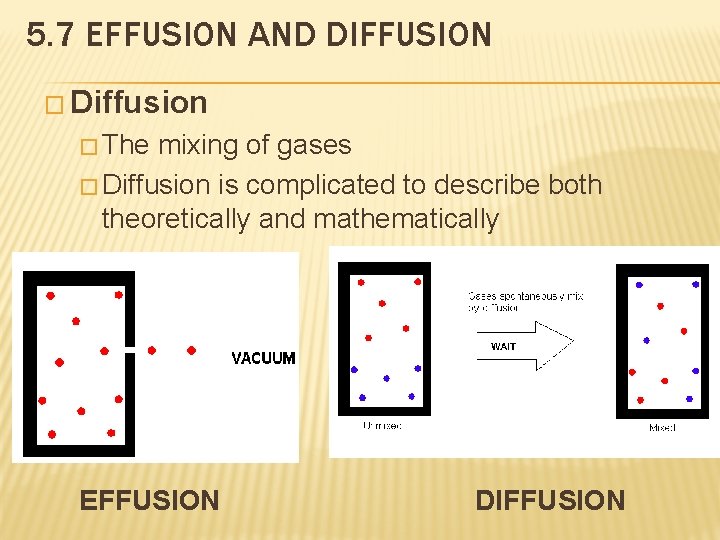
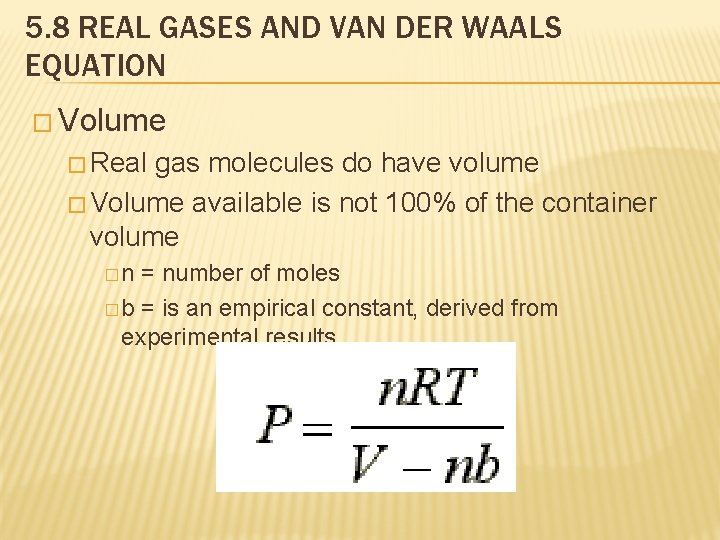
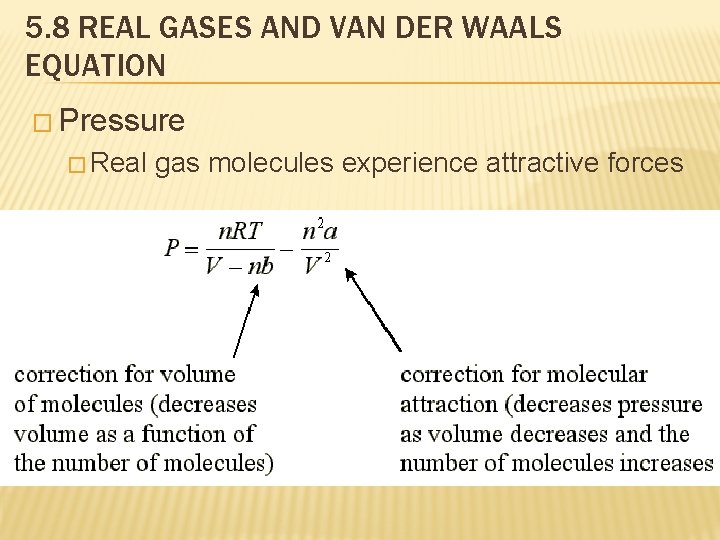
- Slides: 28
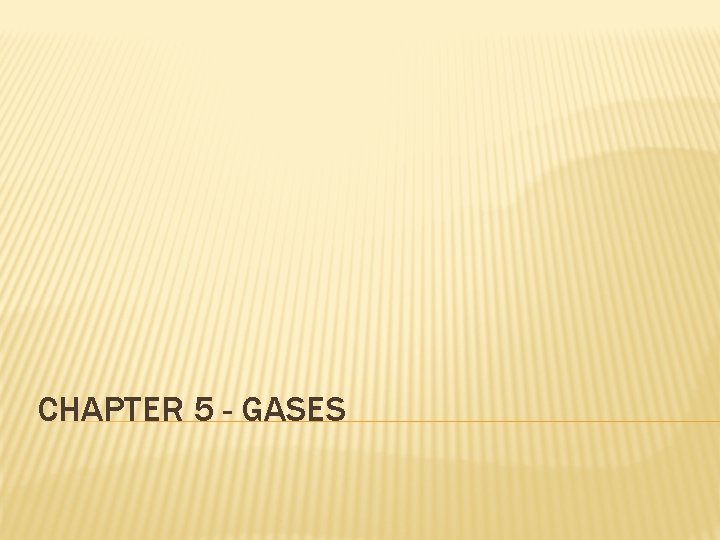
CHAPTER 5 - GASES
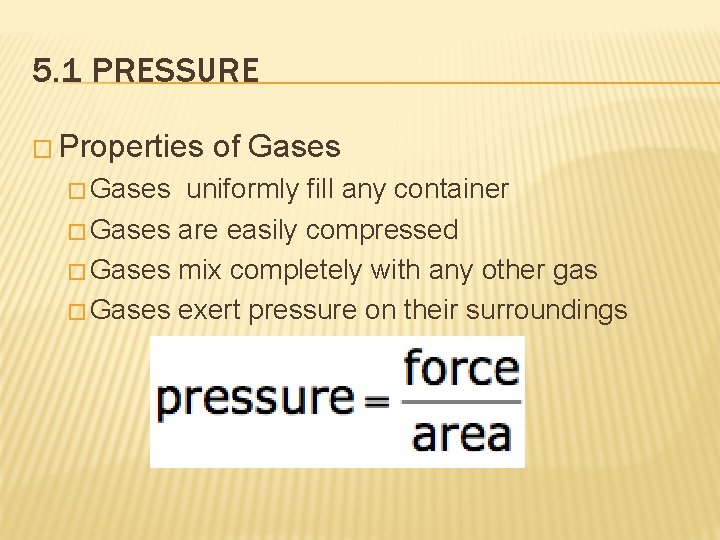
5. 1 PRESSURE � Properties � Gases of Gases uniformly fill any container � Gases are easily compressed � Gases mix completely with any other gas � Gases exert pressure on their surroundings
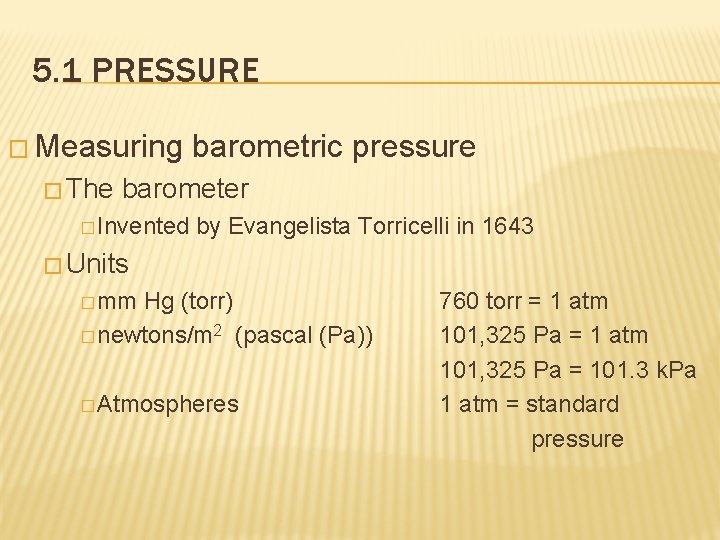
5. 1 PRESSURE � Measuring � The barometric pressure barometer � Invented by Evangelista Torricelli in 1643 � Units � mm Hg (torr) � newtons/m 2 (pascal (Pa)) � Atmospheres 760 torr = 1 atm 101, 325 Pa = 101. 3 k. Pa 1 atm = standard pressure
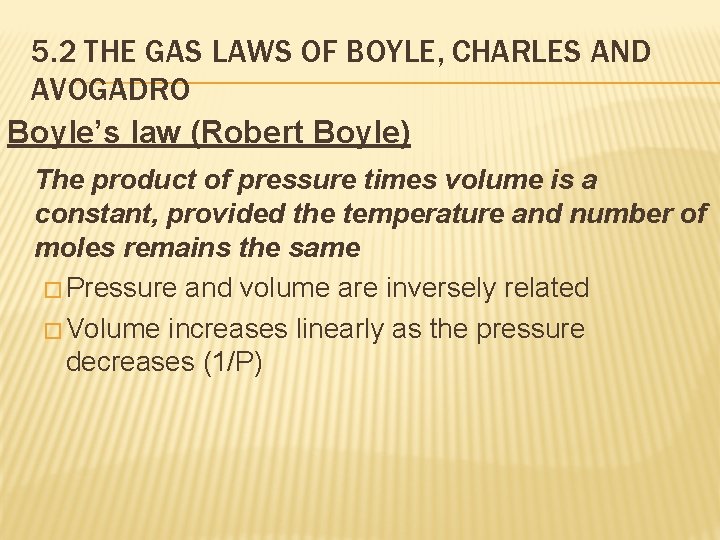
5. 2 THE GAS LAWS OF BOYLE, CHARLES AND AVOGADRO Boyle’s law (Robert Boyle) The product of pressure times volume is a constant, provided the temperature and number of moles remains the same � Pressure and volume are inversely related � Volume increases linearly as the pressure decreases (1/P)
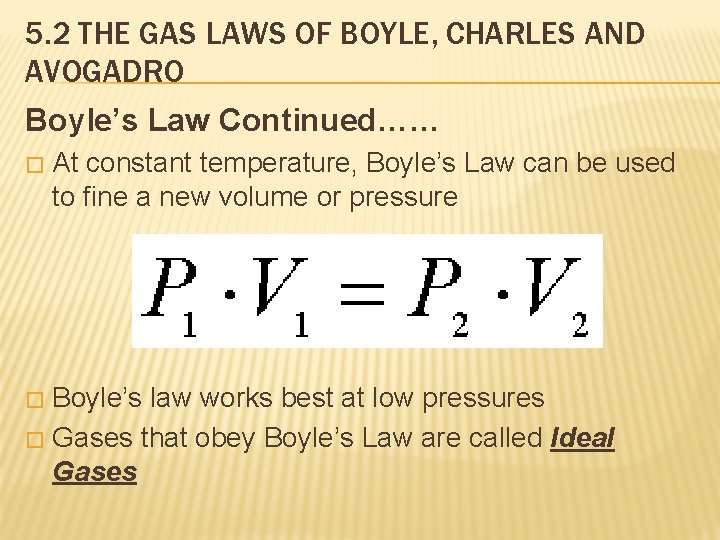
5. 2 THE GAS LAWS OF BOYLE, CHARLES AND AVOGADRO Boyle’s Law Continued…… � At constant temperature, Boyle’s Law can be used to fine a new volume or pressure Boyle’s law works best at low pressures � Gases that obey Boyle’s Law are called Ideal Gases �
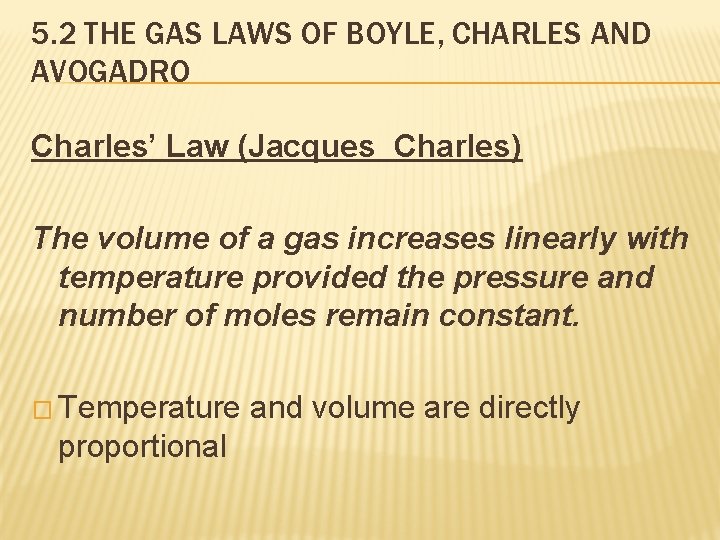
5. 2 THE GAS LAWS OF BOYLE, CHARLES AND AVOGADRO Charles’ Law (Jacques Charles) The volume of a gas increases linearly with temperature provided the pressure and number of moles remain constant. � Temperature proportional and volume are directly
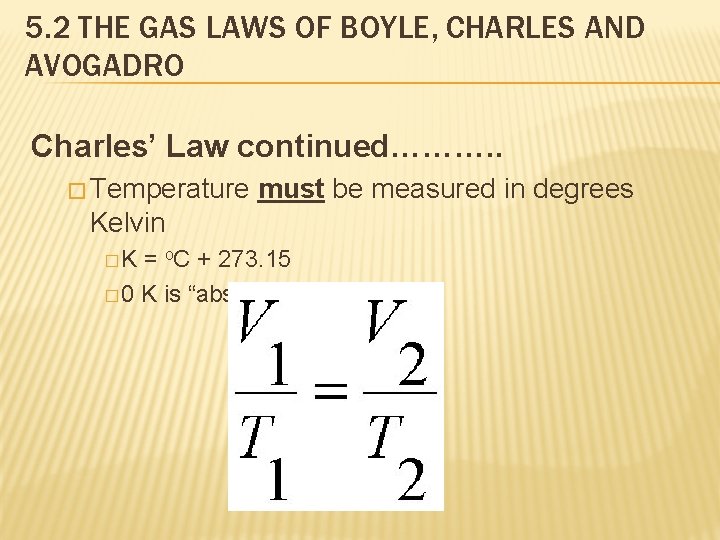
5. 2 THE GAS LAWS OF BOYLE, CHARLES AND AVOGADRO Charles’ Law continued………. . � Temperature must be measured in degrees Kelvin �K = o. C + 273. 15 � 0 K is “absolute zero”
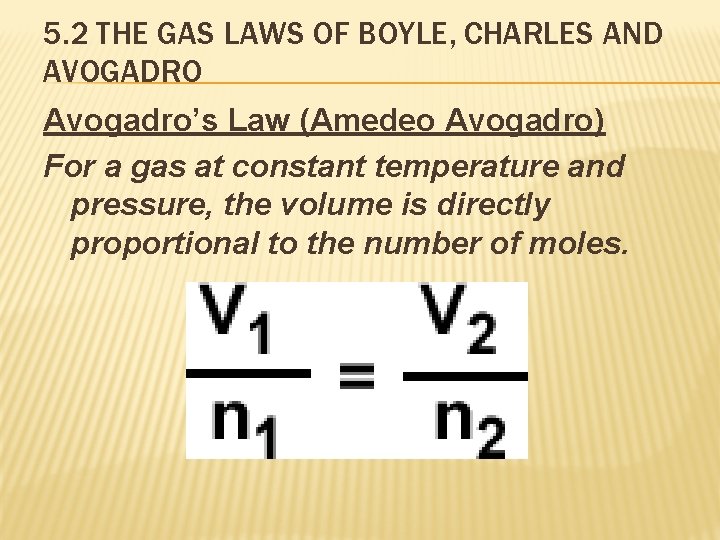
5. 2 THE GAS LAWS OF BOYLE, CHARLES AND AVOGADRO Avogadro’s Law (Amedeo Avogadro) For a gas at constant temperature and pressure, the volume is directly proportional to the number of moles.
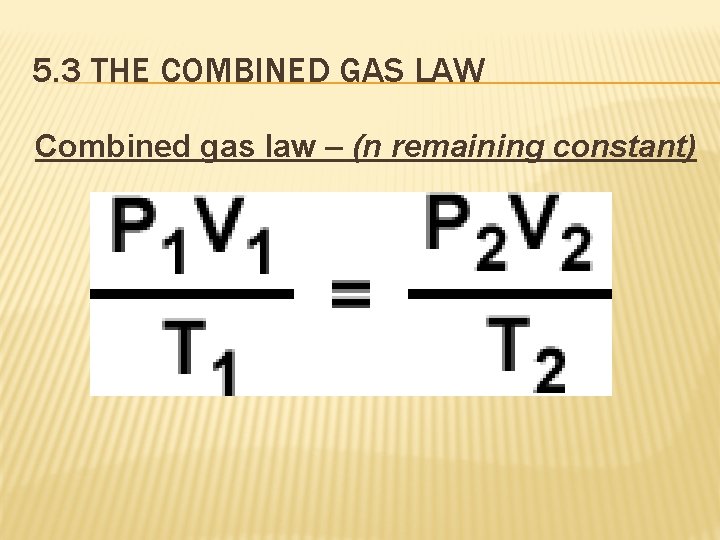
5. 3 THE COMBINED GAS LAW Combined gas law – (n remaining constant)
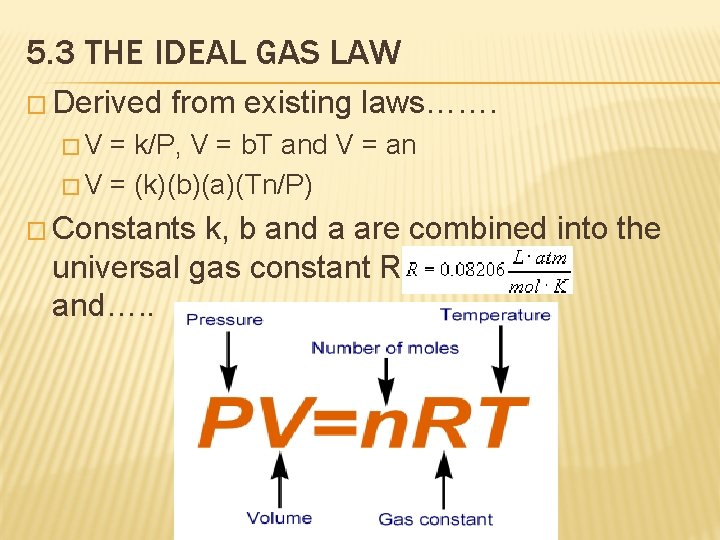
5. 3 THE IDEAL GAS LAW � Derived from existing laws……. �V = k/P, V = b. T and V = an � V = (k)(b)(a)(Tn/P) � Constants k, b and a are combined into the universal gas constant R and…. .
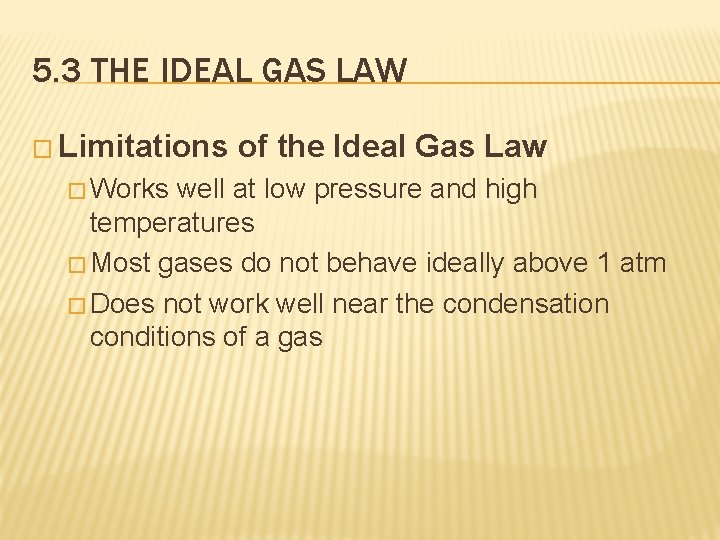
5. 3 THE IDEAL GAS LAW � Limitations � Works of the Ideal Gas Law well at low pressure and high temperatures � Most gases do not behave ideally above 1 atm � Does not work well near the condensation conditions of a gas
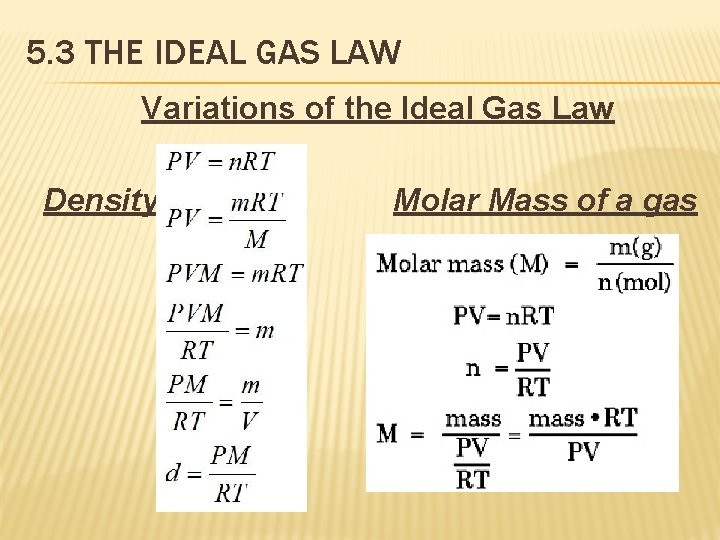
5. 3 THE IDEAL GAS LAW Variations of the Ideal Gas Law Density Molar Mass of a gas
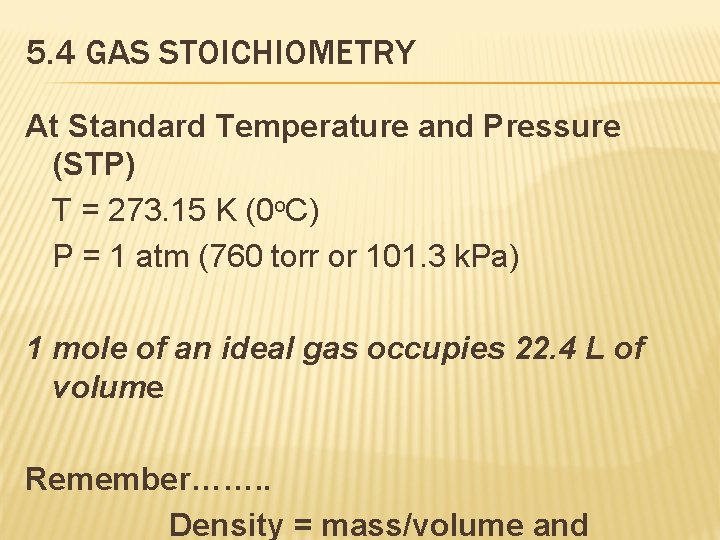
5. 4 GAS STOICHIOMETRY At Standard Temperature and Pressure (STP) T = 273. 15 K (0 o. C) P = 1 atm (760 torr or 101. 3 k. Pa) 1 mole of an ideal gas occupies 22. 4 L of volume Remember……. . Density = mass/volume and
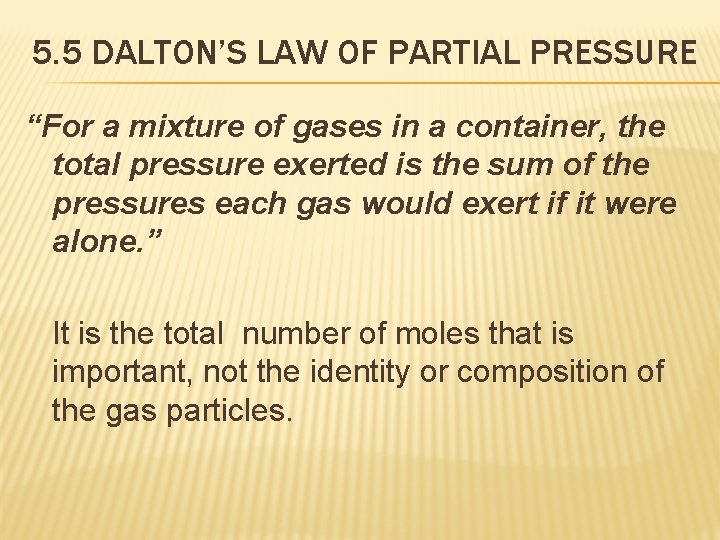
5. 5 DALTON’S LAW OF PARTIAL PRESSURE “For a mixture of gases in a container, the total pressure exerted is the sum of the pressures each gas would exert if it were alone. ” It is the total number of moles that is important, not the identity or composition of the gas particles.
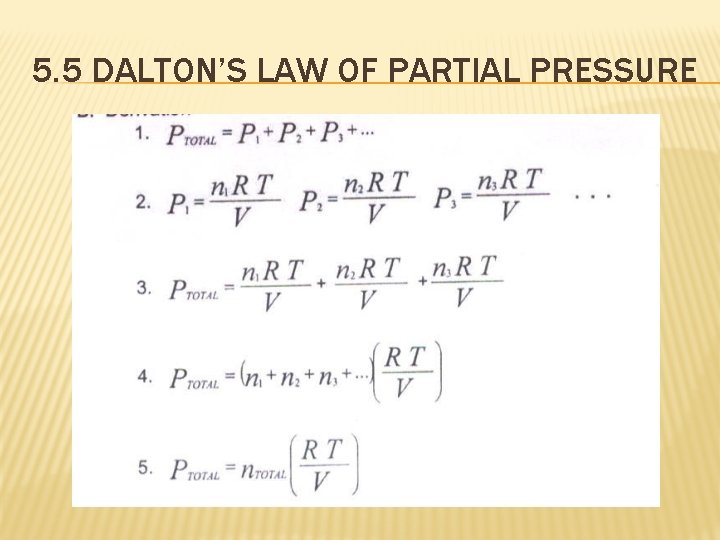
5. 5 DALTON’S LAW OF PARTIAL PRESSURE
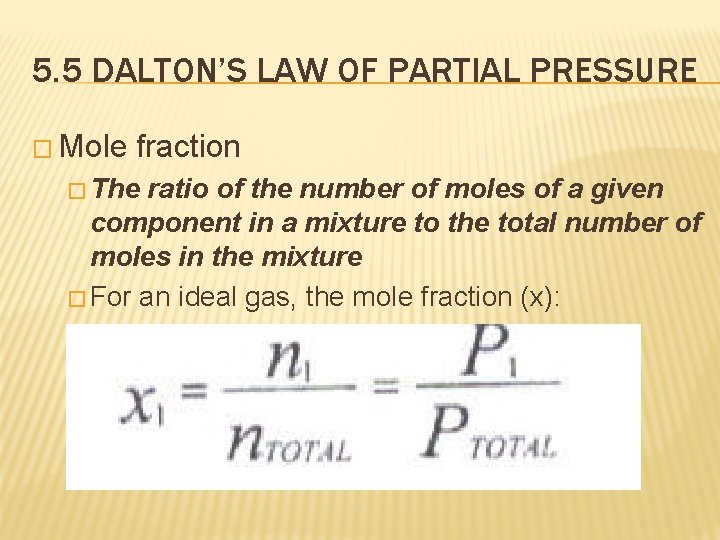
5. 5 DALTON’S LAW OF PARTIAL PRESSURE � Mole fraction � The ratio of the number of moles of a given component in a mixture to the total number of moles in the mixture � For an ideal gas, the mole fraction (x):
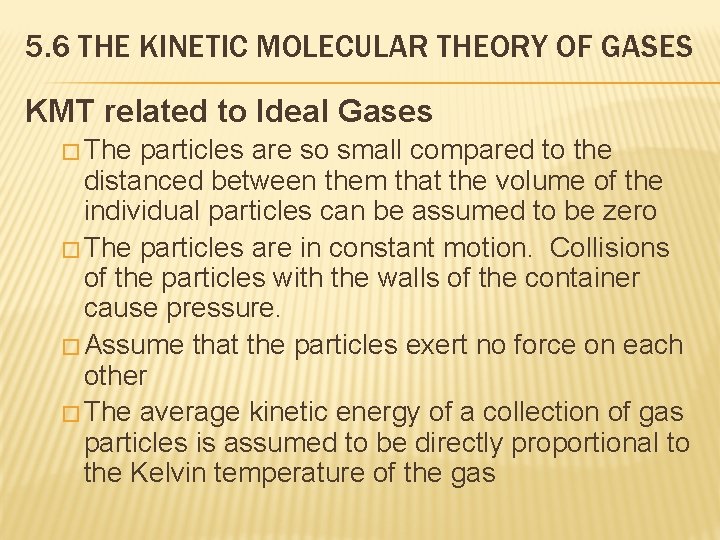
5. 6 THE KINETIC MOLECULAR THEORY OF GASES KMT related to Ideal Gases � The particles are so small compared to the distanced between them that the volume of the individual particles can be assumed to be zero � The particles are in constant motion. Collisions of the particles with the walls of the container cause pressure. � Assume that the particles exert no force on each other � The average kinetic energy of a collection of gas particles is assumed to be directly proportional to the Kelvin temperature of the gas
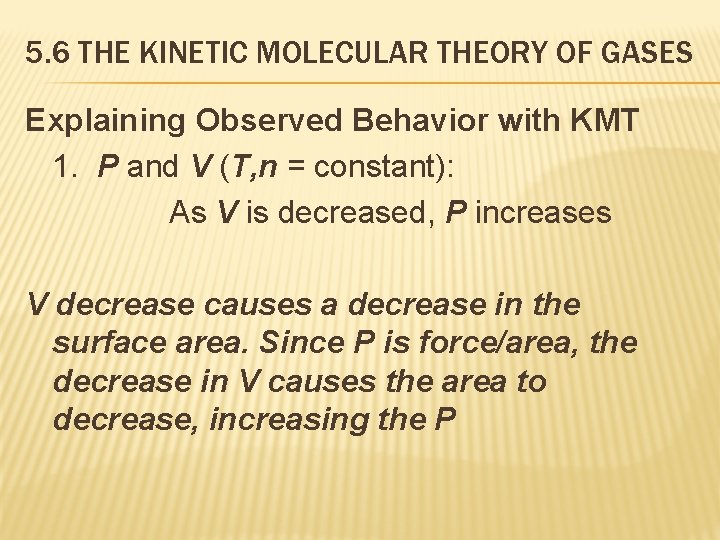
5. 6 THE KINETIC MOLECULAR THEORY OF GASES Explaining Observed Behavior with KMT 1. P and V (T, n = constant): As V is decreased, P increases V decrease causes a decrease in the surface area. Since P is force/area, the decrease in V causes the area to decrease, increasing the P
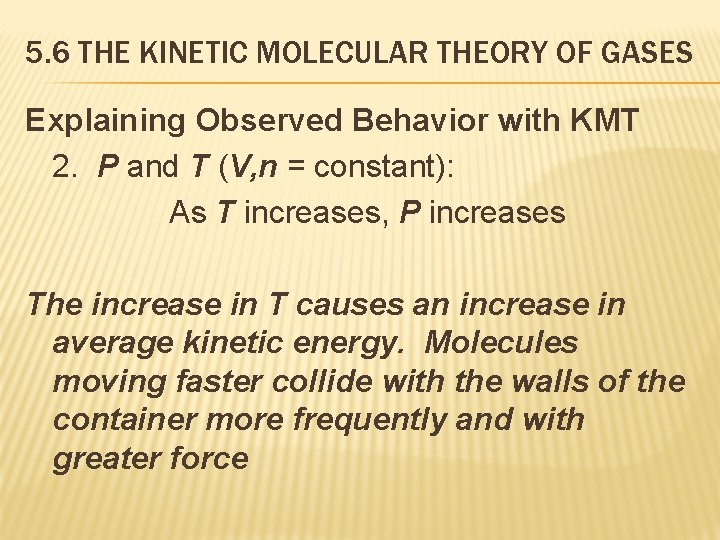
5. 6 THE KINETIC MOLECULAR THEORY OF GASES Explaining Observed Behavior with KMT 2. P and T (V, n = constant): As T increases, P increases The increase in T causes an increase in average kinetic energy. Molecules moving faster collide with the walls of the container more frequently and with greater force
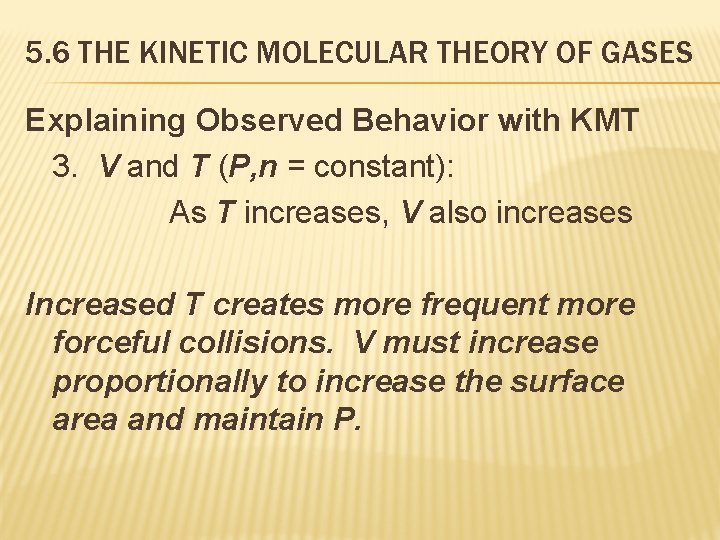
5. 6 THE KINETIC MOLECULAR THEORY OF GASES Explaining Observed Behavior with KMT 3. V and T (P, n = constant): As T increases, V also increases Increased T creates more frequent more forceful collisions. V must increase proportionally to increase the surface area and maintain P.
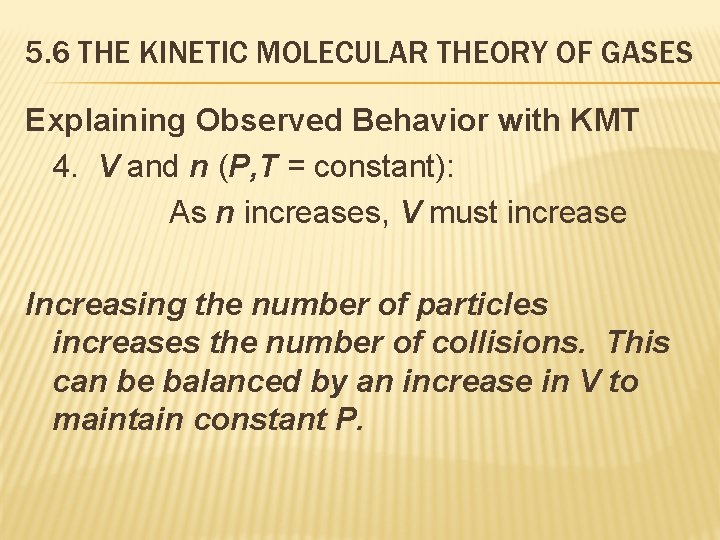
5. 6 THE KINETIC MOLECULAR THEORY OF GASES Explaining Observed Behavior with KMT 4. V and n (P, T = constant): As n increases, V must increase Increasing the number of particles increases the number of collisions. This can be balanced by an increase in V to maintain constant P.
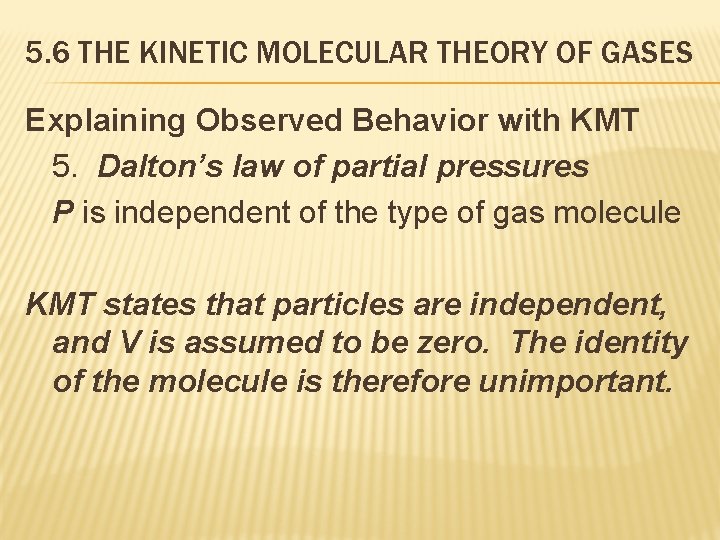
5. 6 THE KINETIC MOLECULAR THEORY OF GASES Explaining Observed Behavior with KMT 5. Dalton’s law of partial pressures P is independent of the type of gas molecule KMT states that particles are independent, and V is assumed to be zero. The identity of the molecule is therefore unimportant.
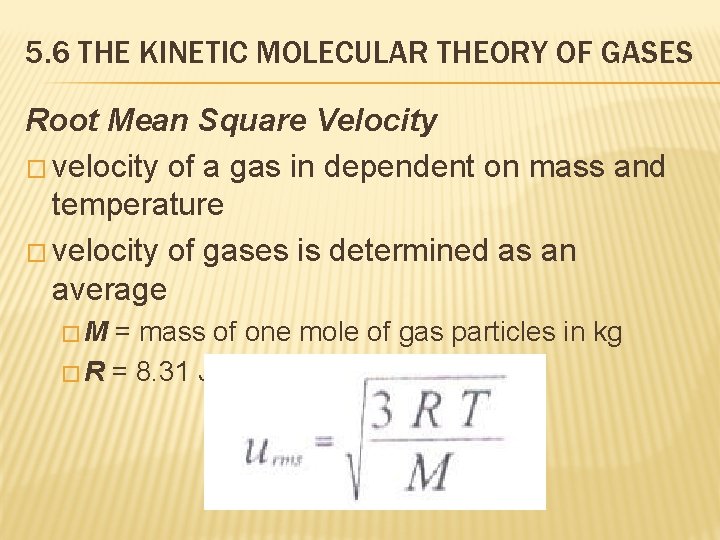
5. 6 THE KINETIC MOLECULAR THEORY OF GASES Root Mean Square Velocity � velocity of a gas in dependent on mass and temperature � velocity of gases is determined as an average �M = mass of one mole of gas particles in kg � R = 8. 31 J/K · mol
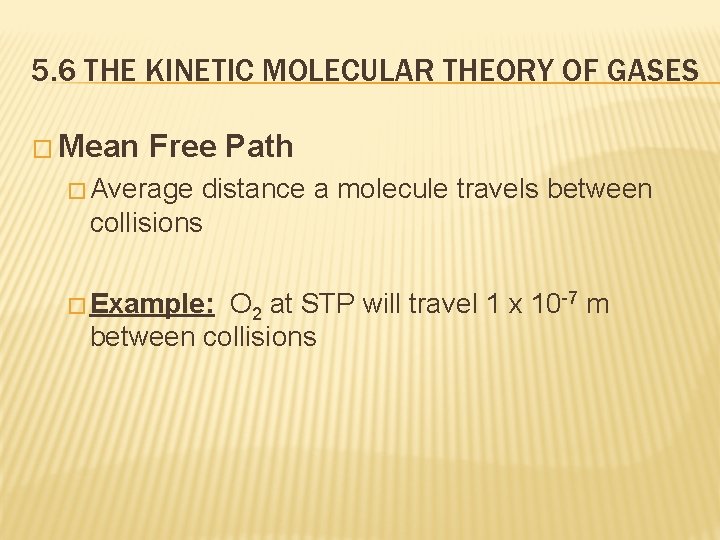
5. 6 THE KINETIC MOLECULAR THEORY OF GASES � Mean Free Path � Average distance a molecule travels between collisions � Example: O 2 at STP will travel 1 x 10 -7 m between collisions
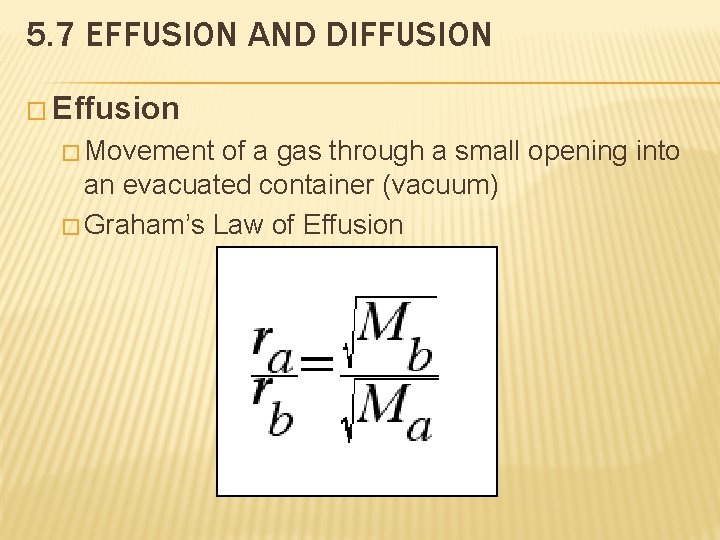
5. 7 EFFUSION AND DIFFUSION � Effusion � Movement of a gas through a small opening into an evacuated container (vacuum) � Graham’s Law of Effusion
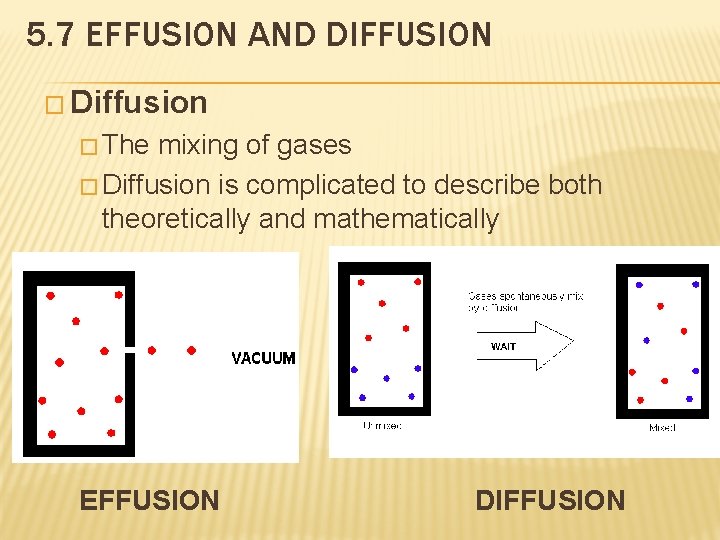
5. 7 EFFUSION AND DIFFUSION � Diffusion � The mixing of gases � Diffusion is complicated to describe both theoretically and mathematically EFFUSION DIFFUSION
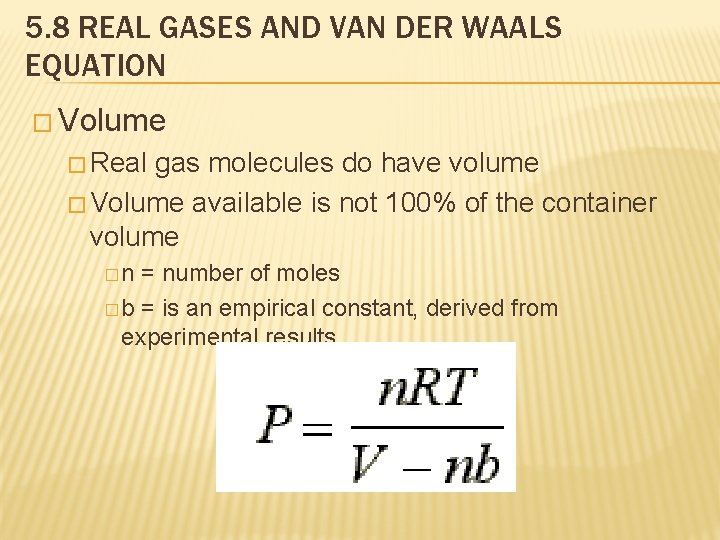
5. 8 REAL GASES AND VAN DER WAALS EQUATION � Volume � Real gas molecules do have volume � Volume available is not 100% of the container volume �n = number of moles � b = is an empirical constant, derived from experimental results
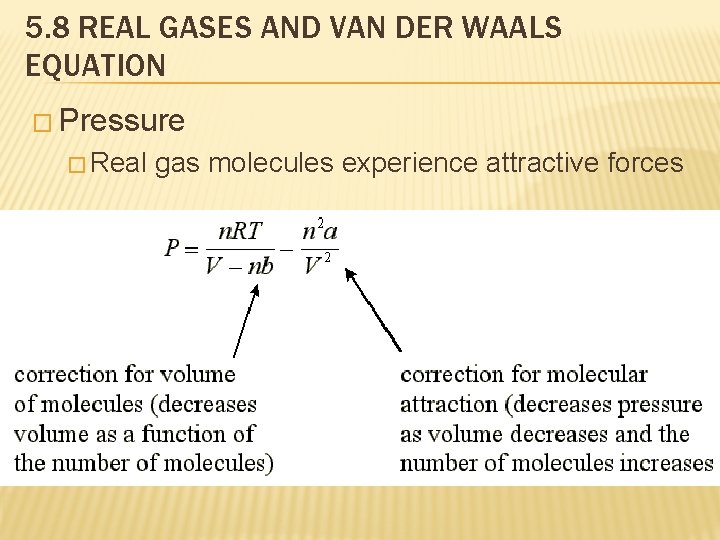
5. 8 REAL GASES AND VAN DER WAALS EQUATION � Pressure � Real gas molecules experience attractive forces
How does a gas exert pressure
Do gases exert pressure on whatever surrounds them
Solids liquids and gases section 2 properties of fluids
Solid
Properties of gas
Four properties of gases
5 properties of gases
Properties of gases
Properties of noble gas
What are the different properties of gas?
Gases have low densities
What is noble gas
Properties of solids liquids and gases
State avogadro's law
Properties of gases
List 2 of the important properties of gases
Patella baja
Pressure support vs pressure control
Pressure mapping for pressure ulcers
Intrapleural pressure
Oncotic vs osmotic
Partial pressure
Intrapulmonary pressure
Metamorphic grade
Pressure support vs pressure control
Dynamic pressure formula
Factors affecting gfr
Oncotic pressure
Oncoric pressure