CHEMISTRY A MOLECULAR APPROACH NIVALDO J TRO Westmont
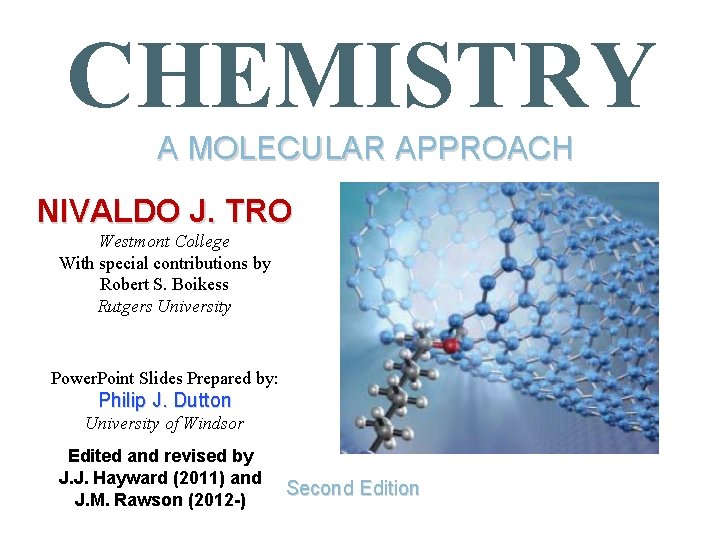
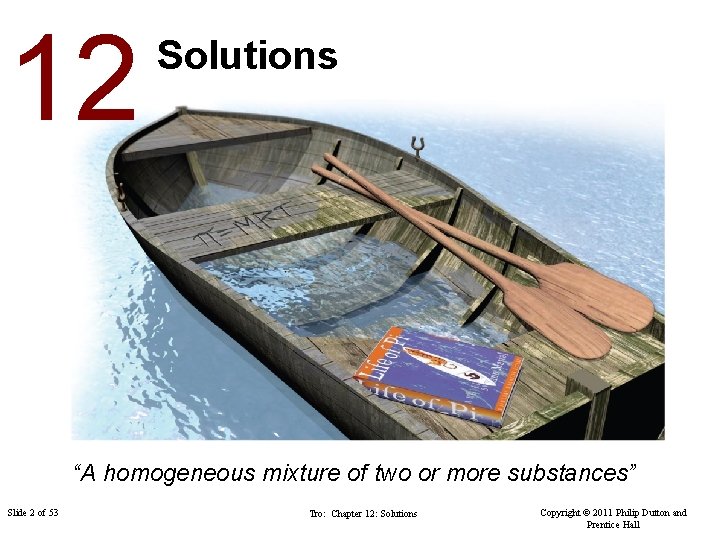
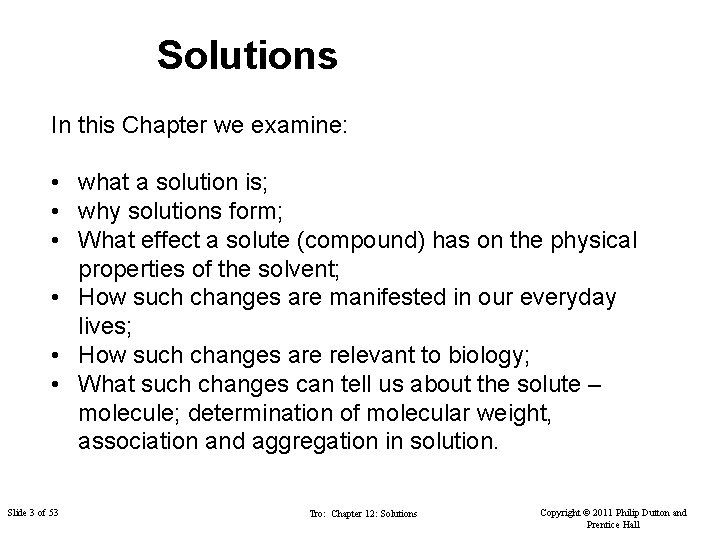
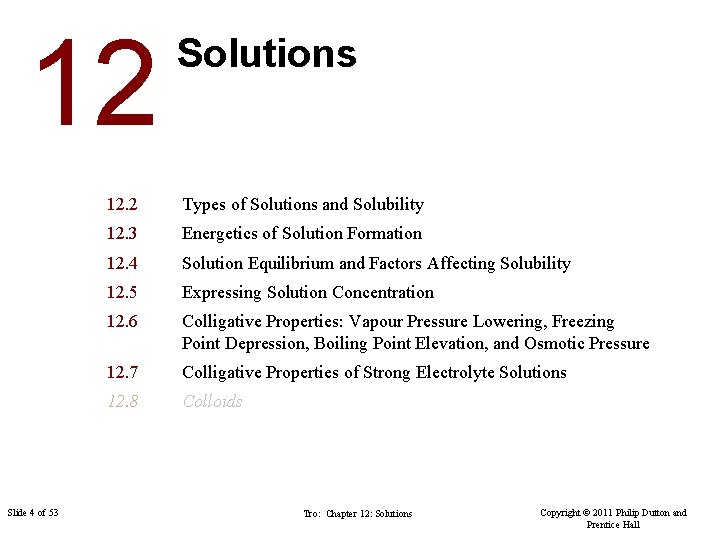
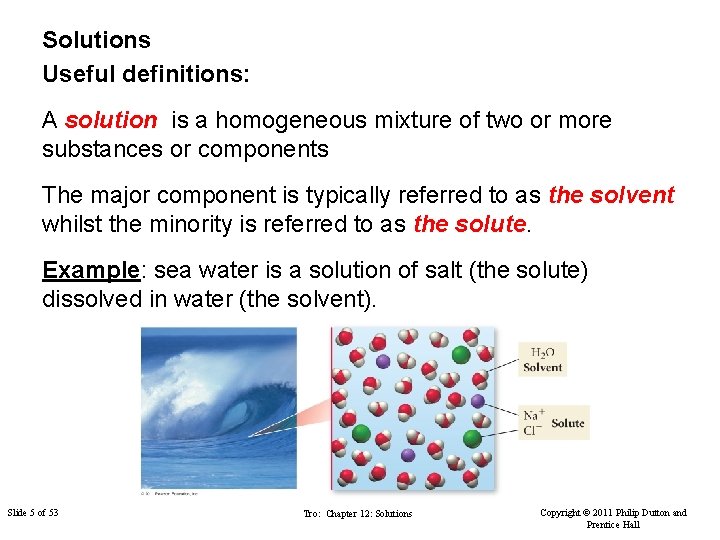
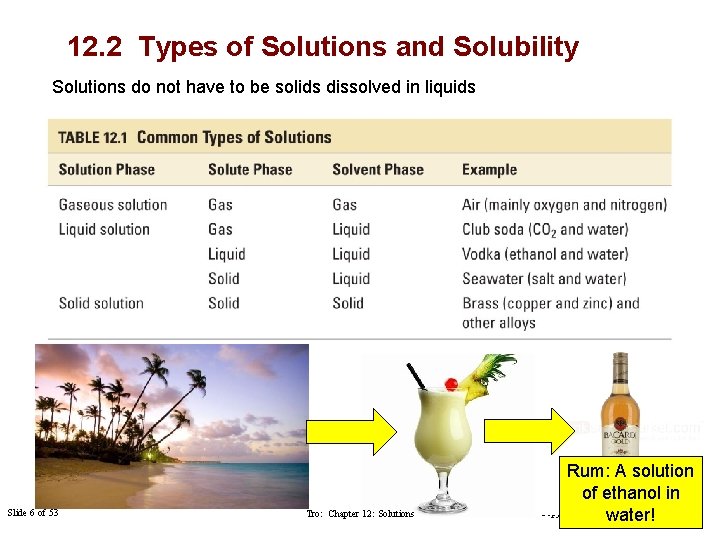
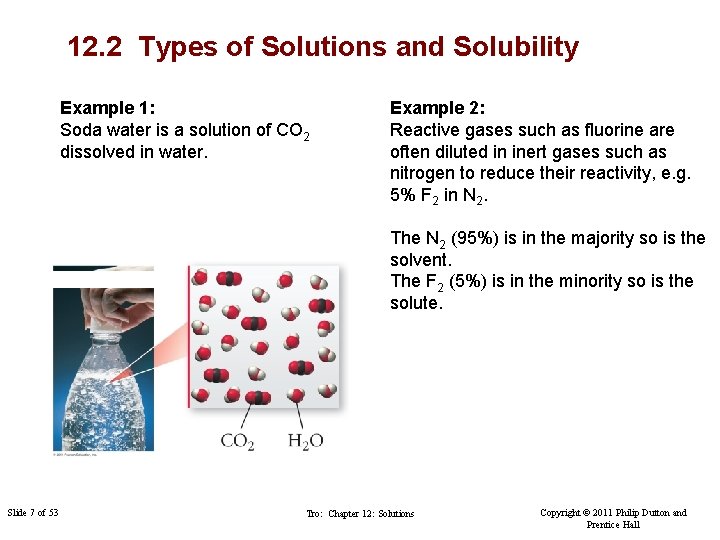
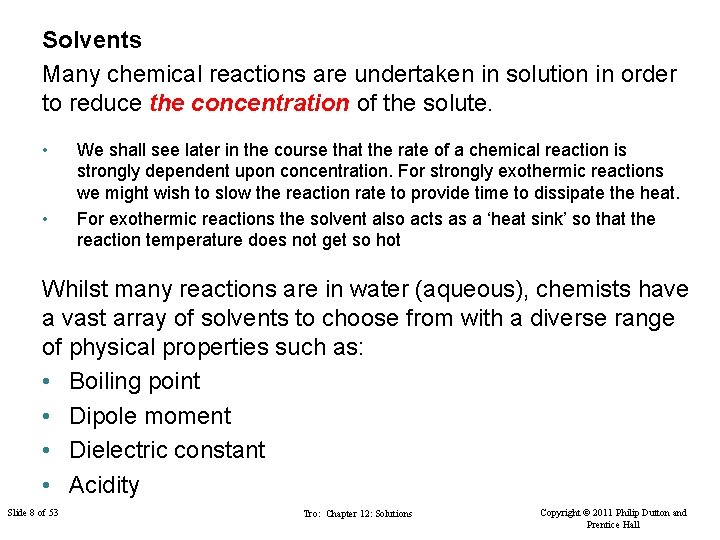
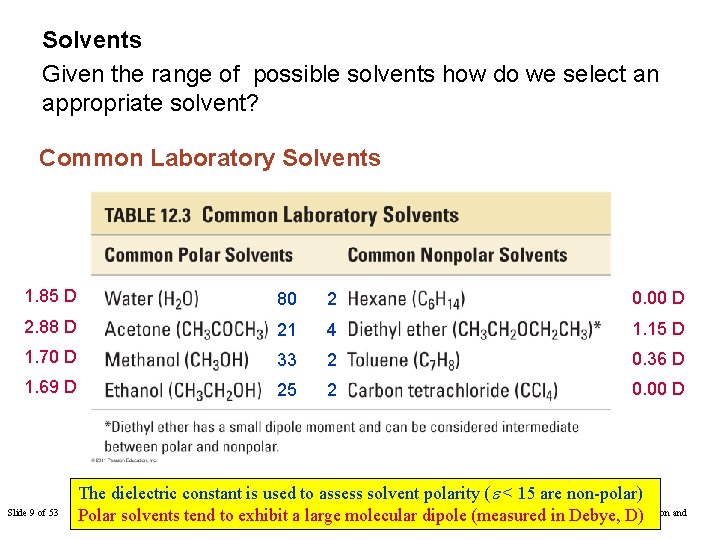
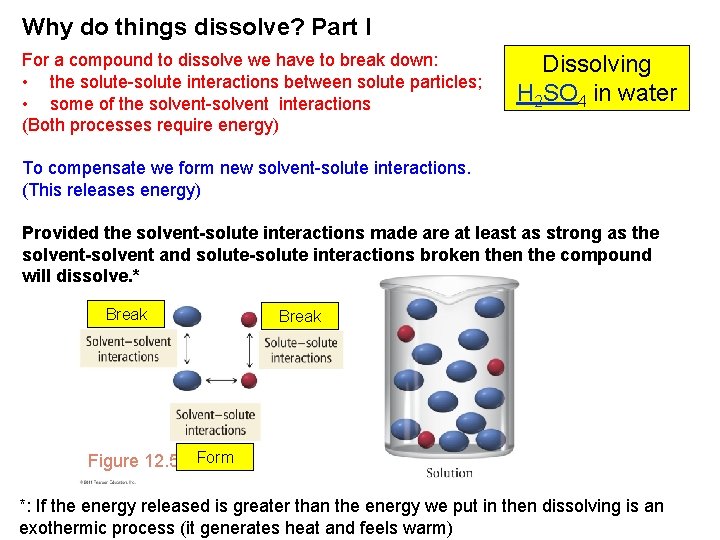
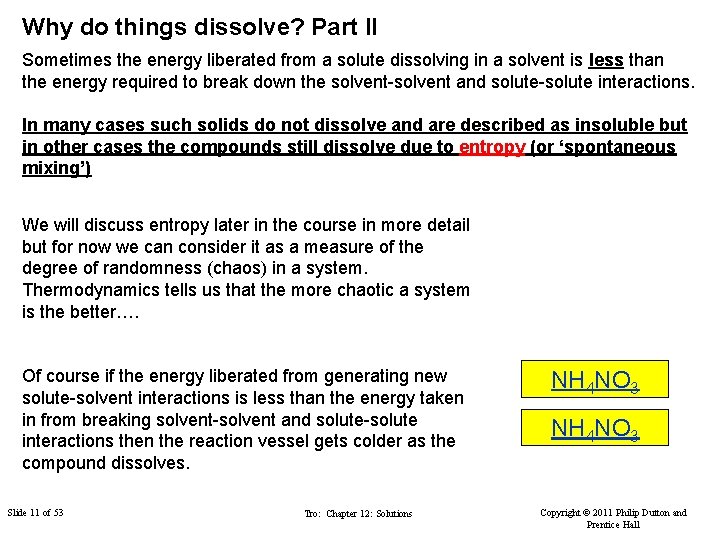
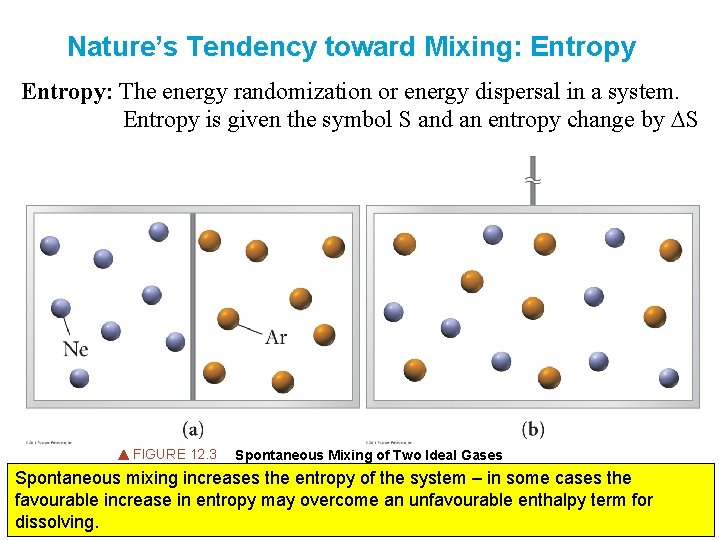
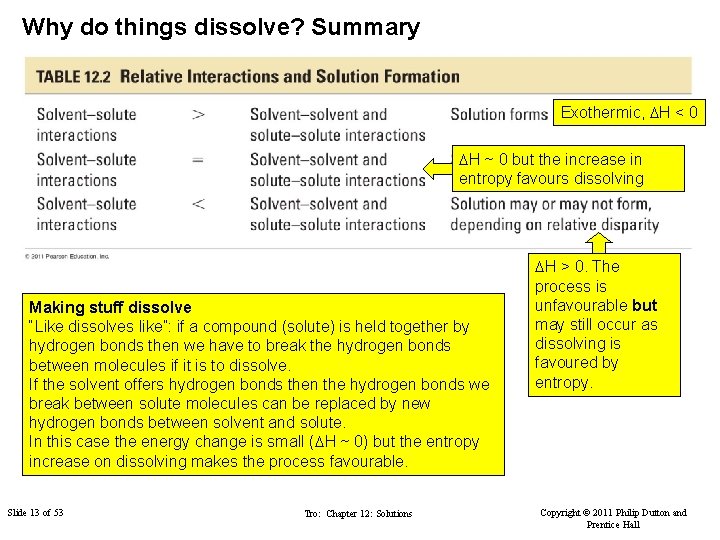
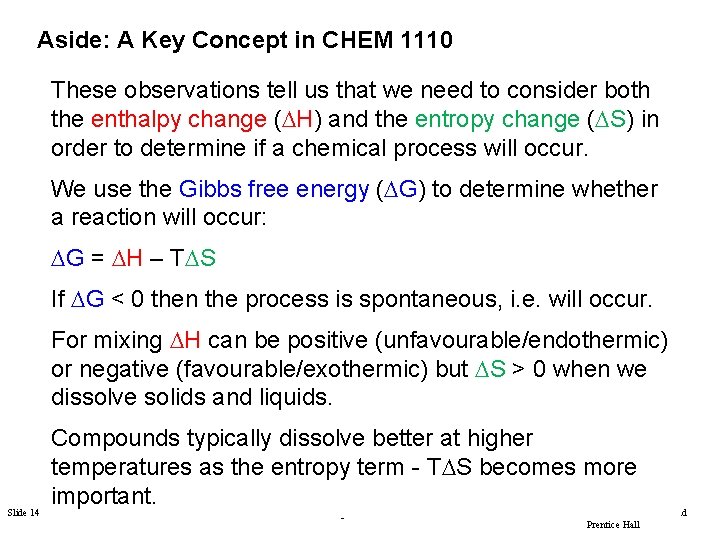
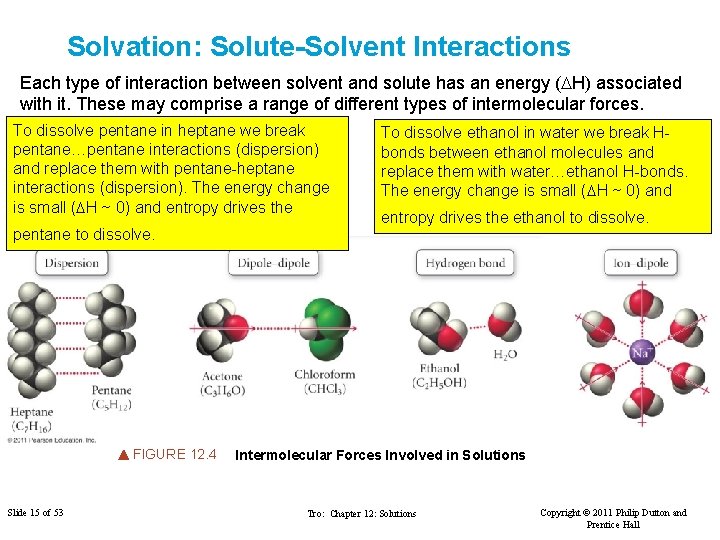
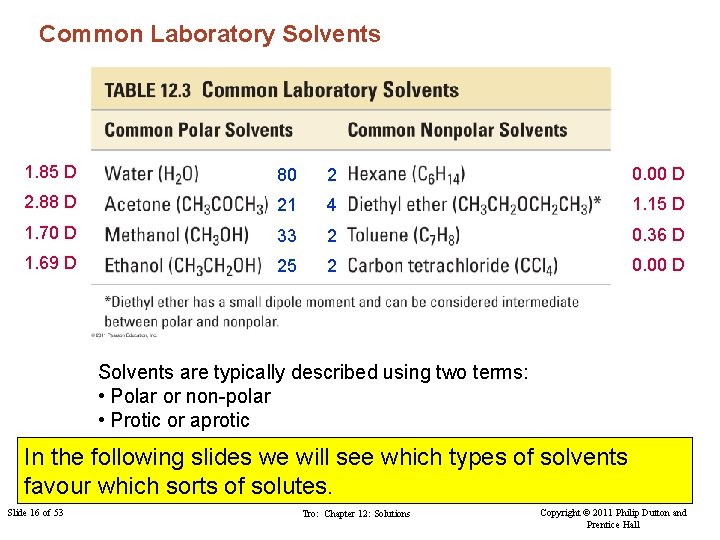
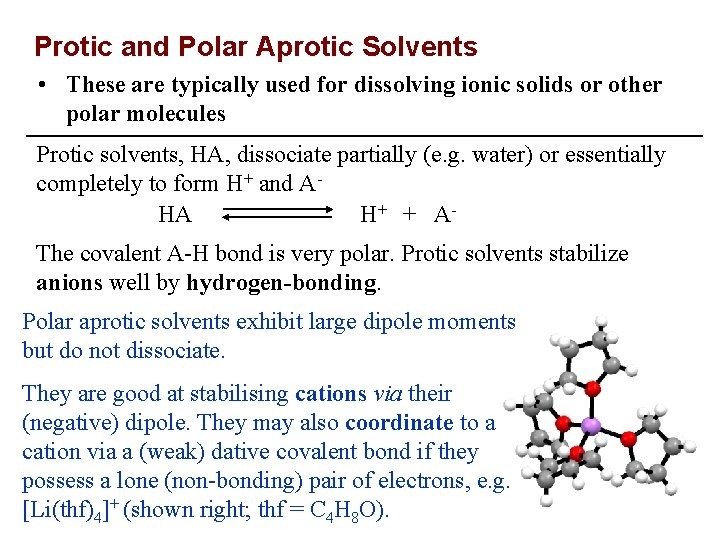
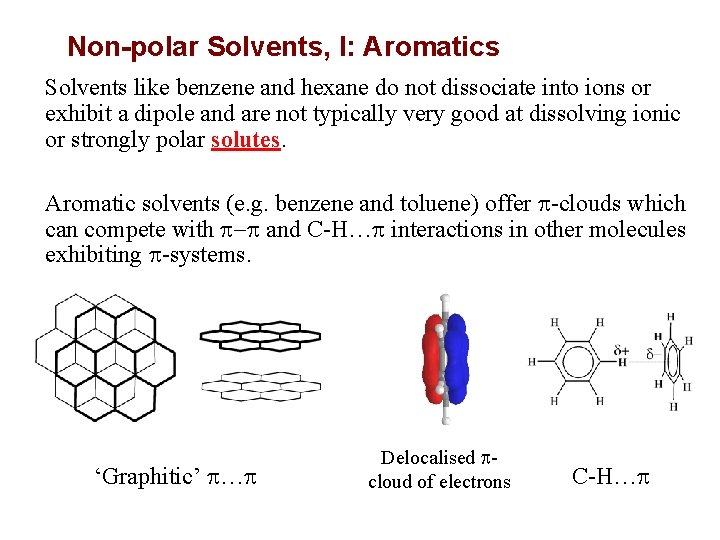
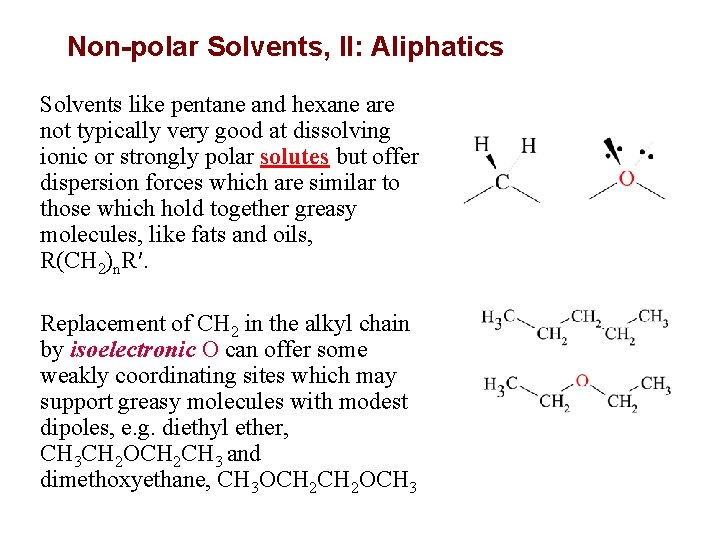
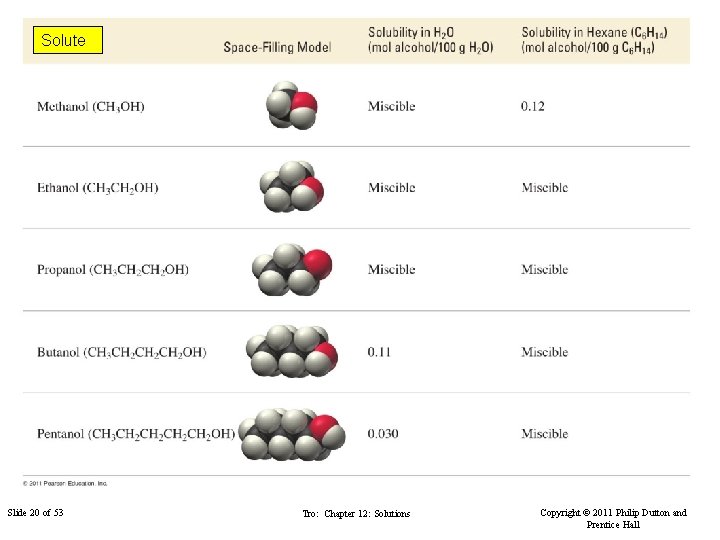
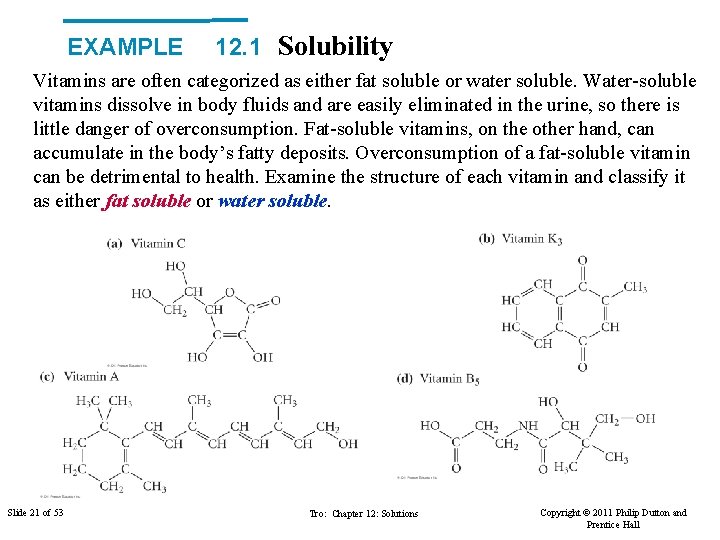
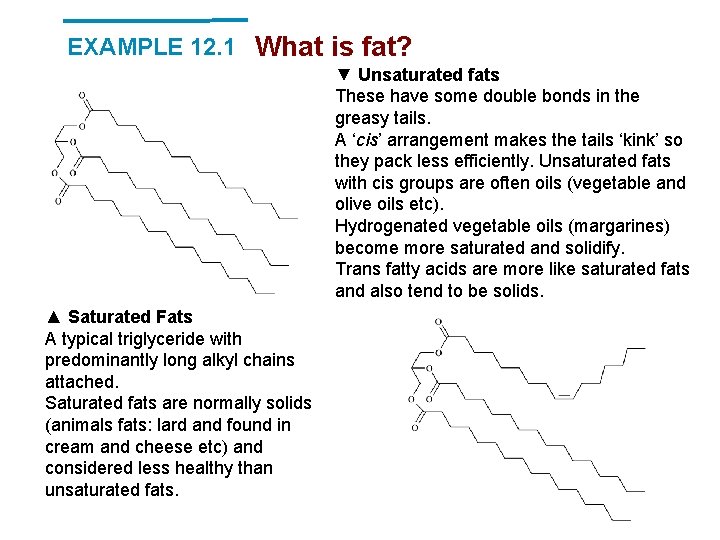
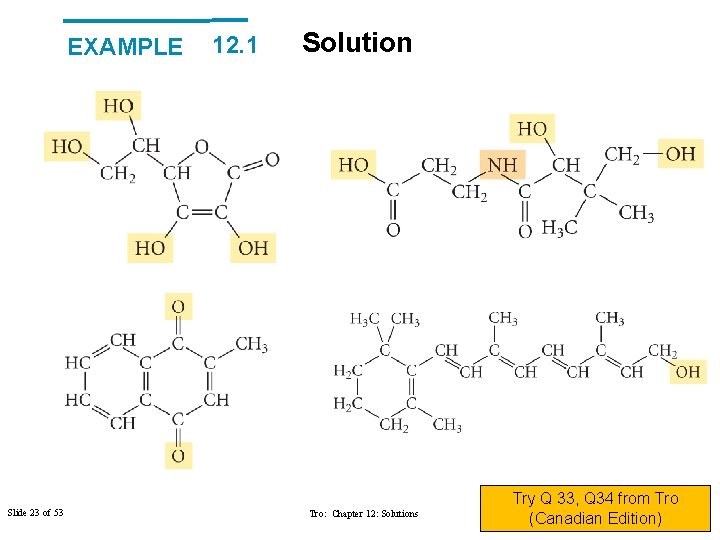
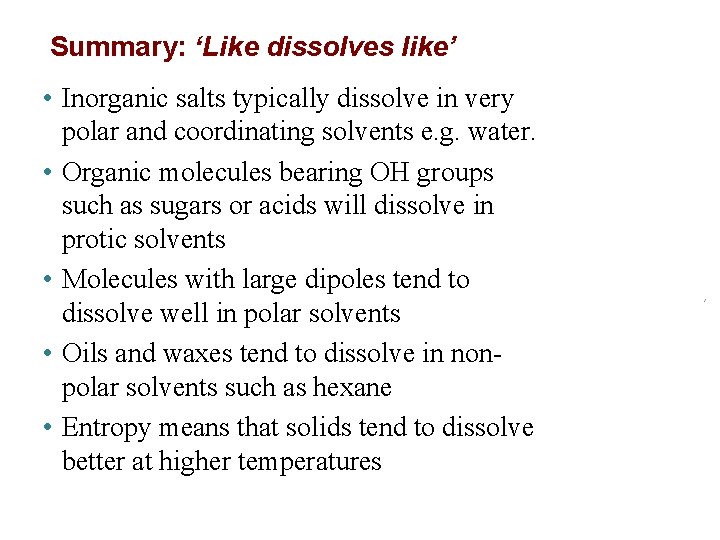
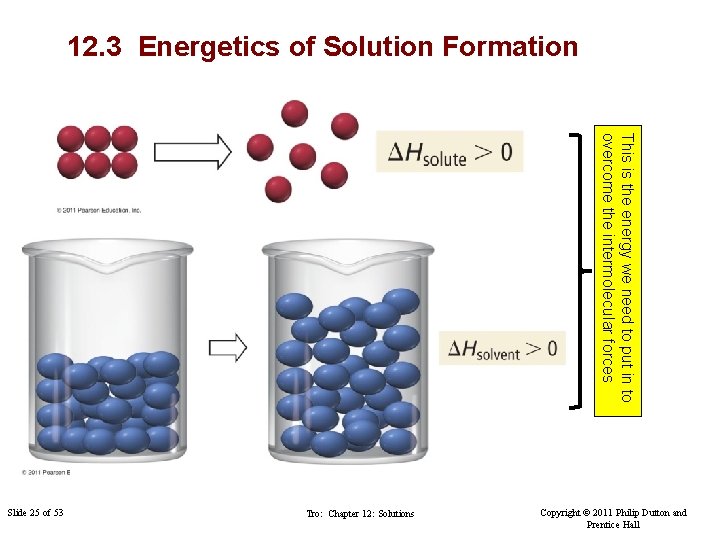
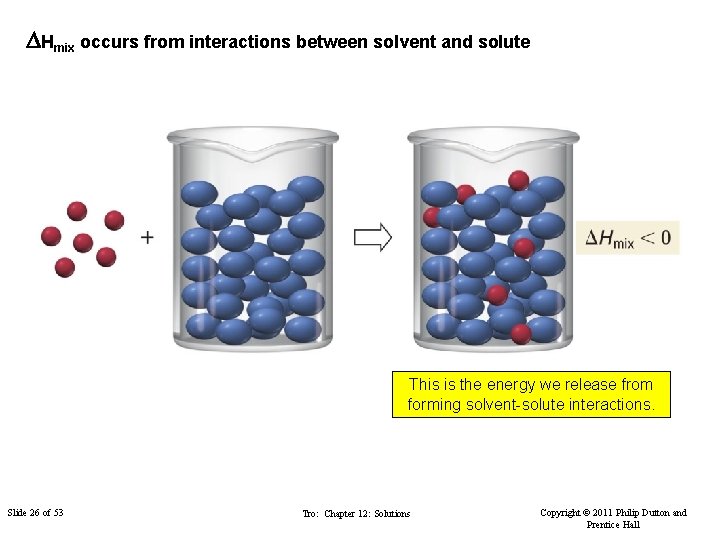
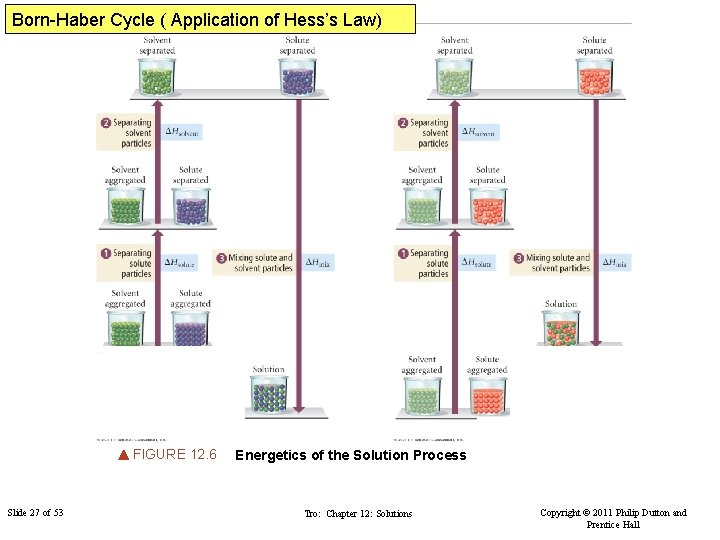
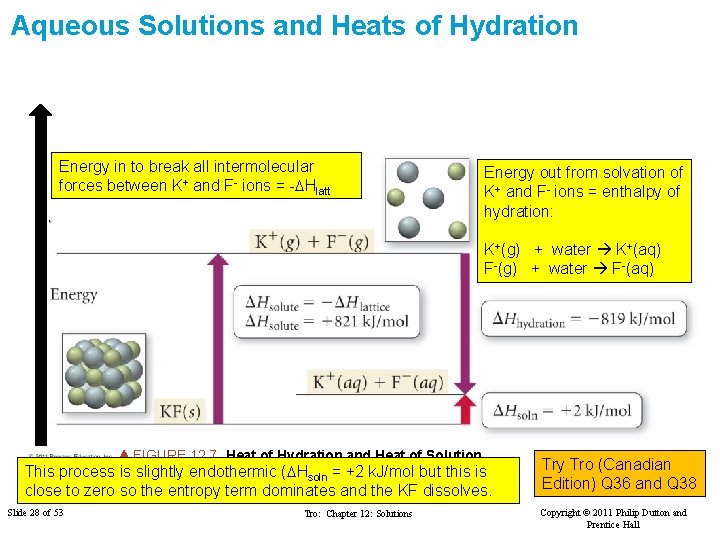
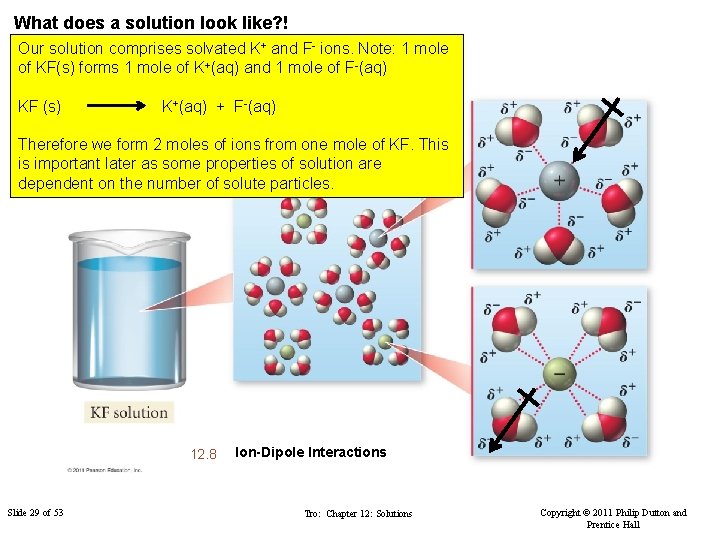
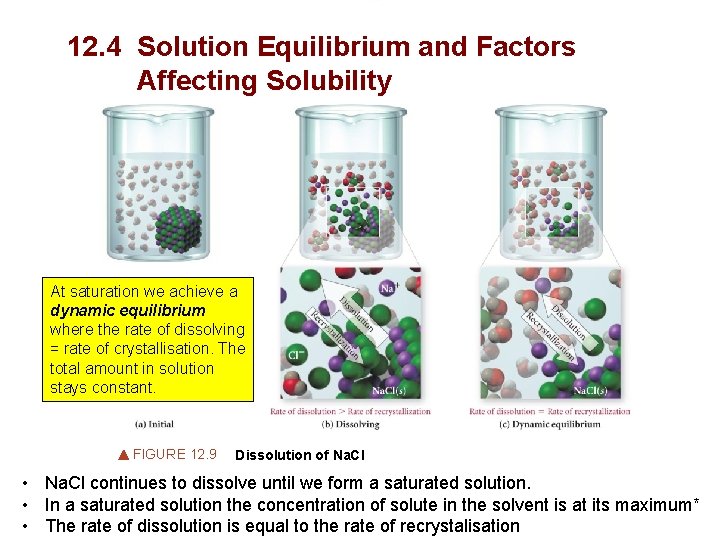
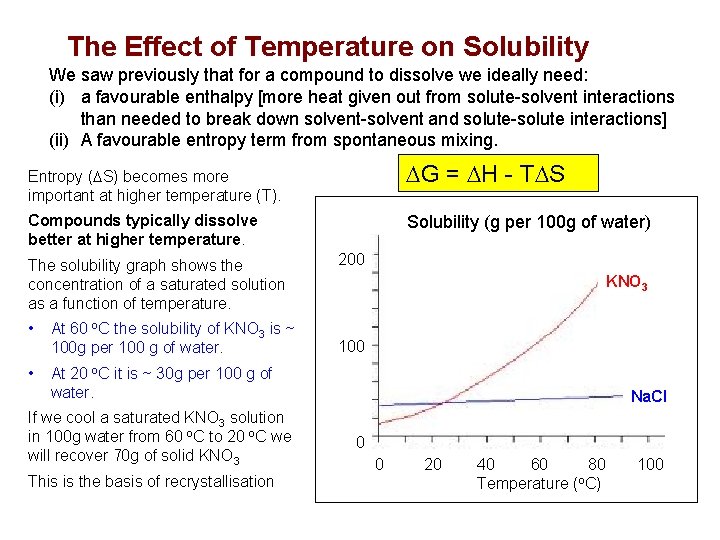
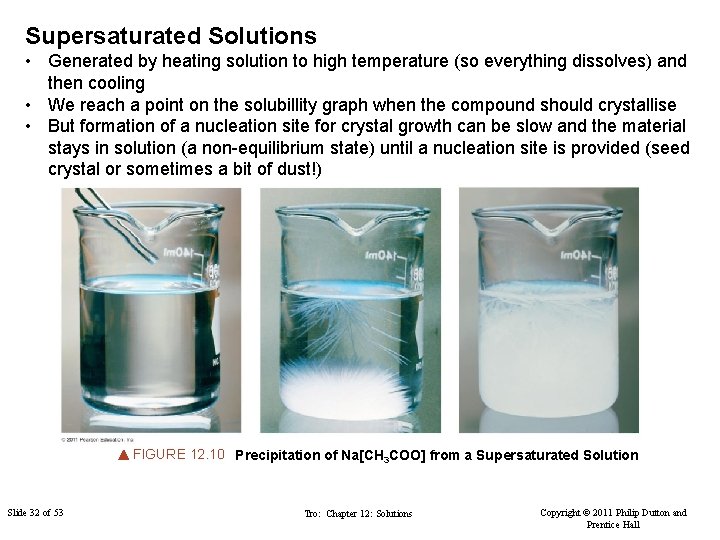
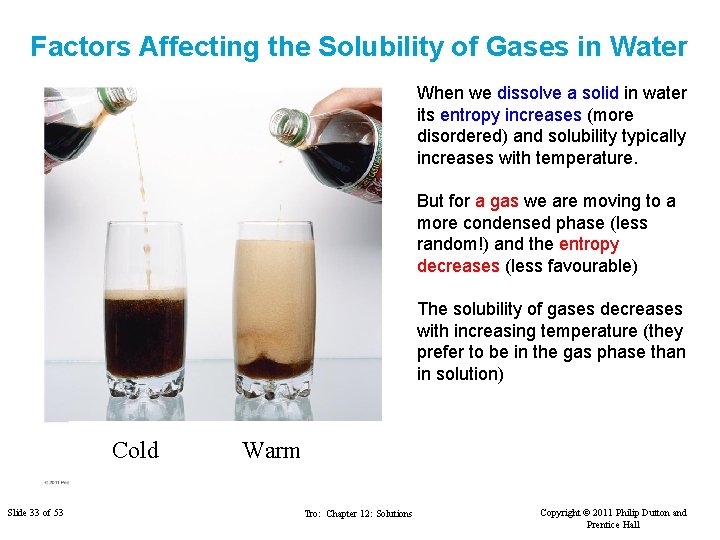
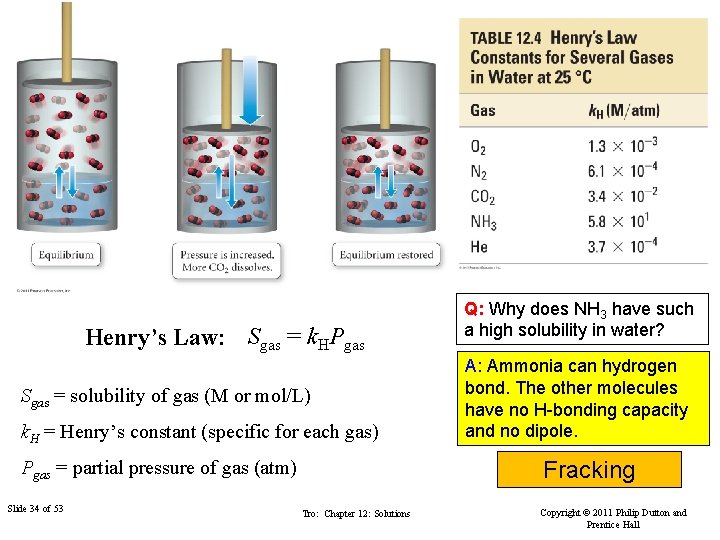
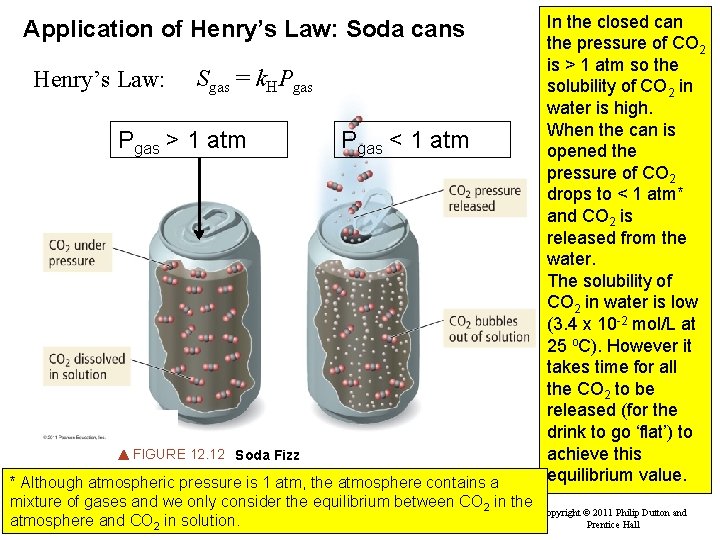
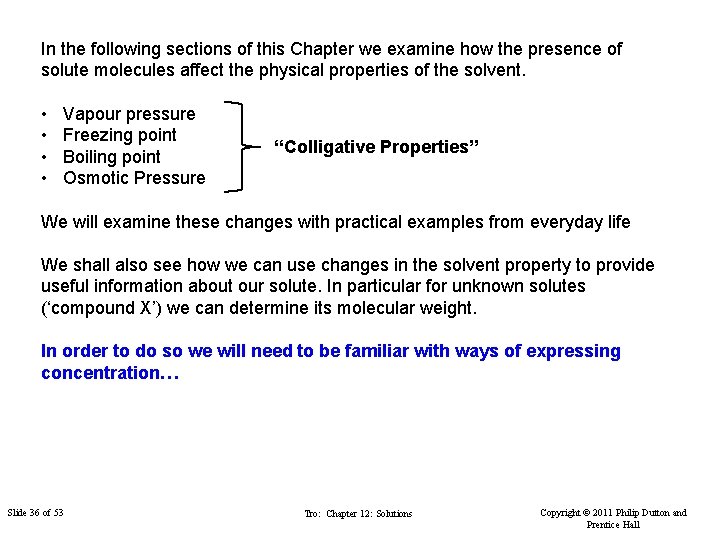
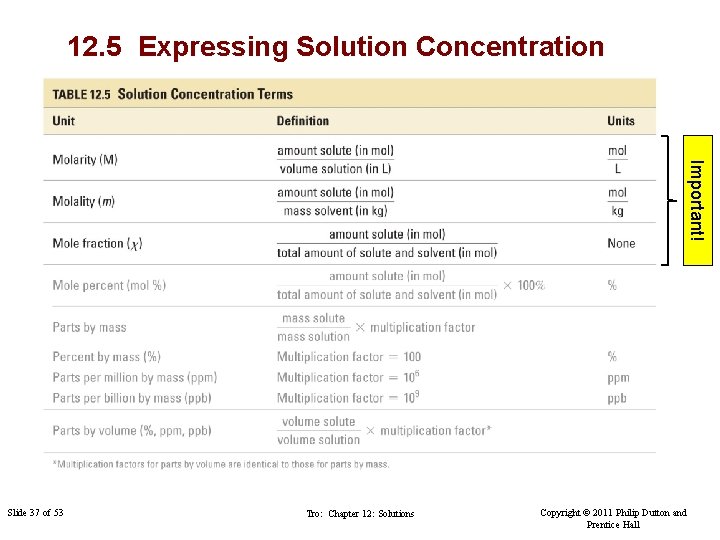
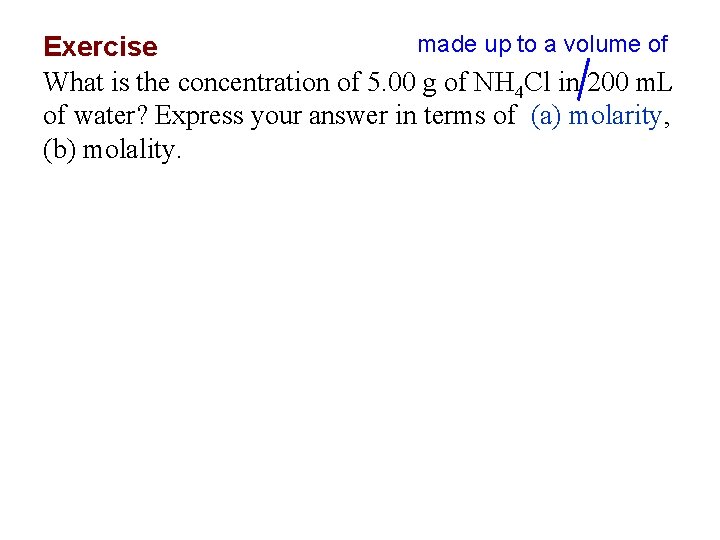
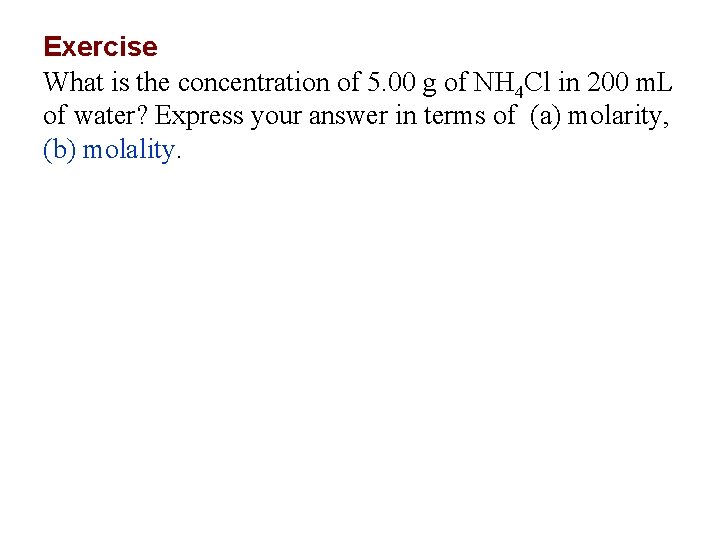
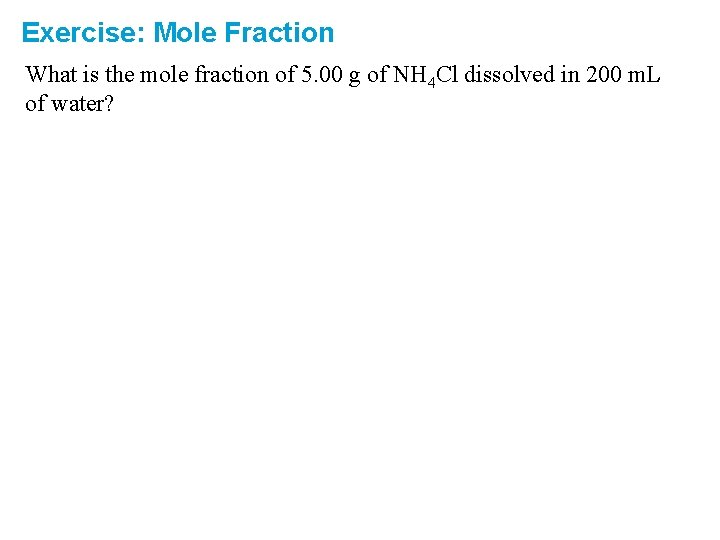
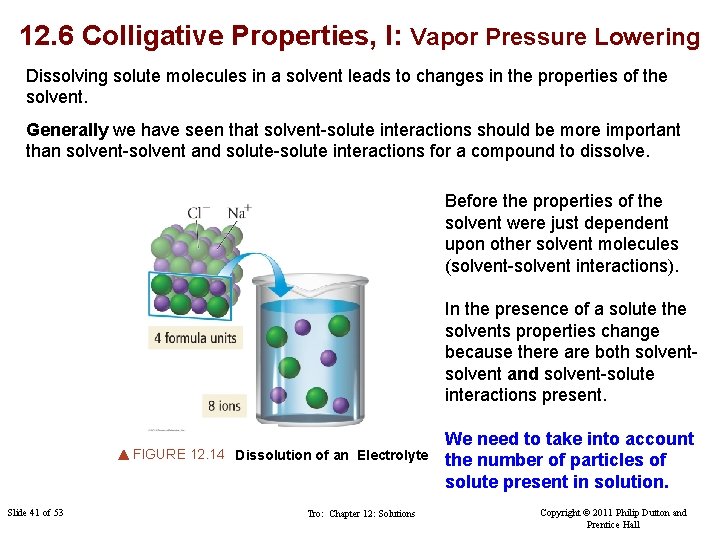
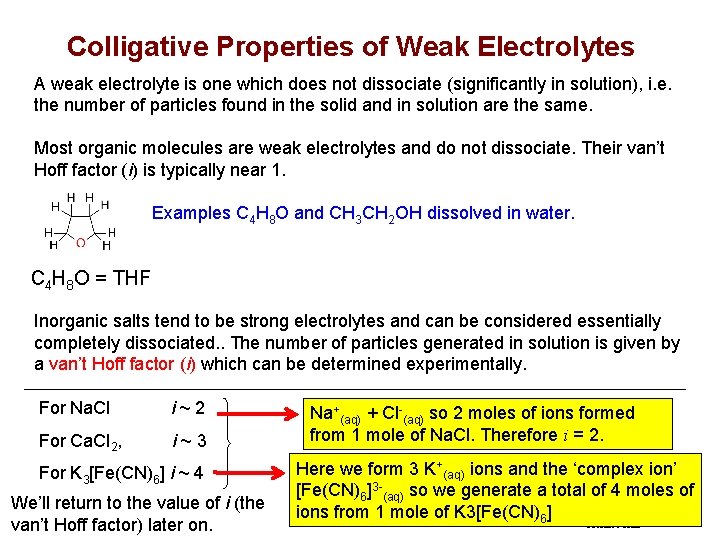
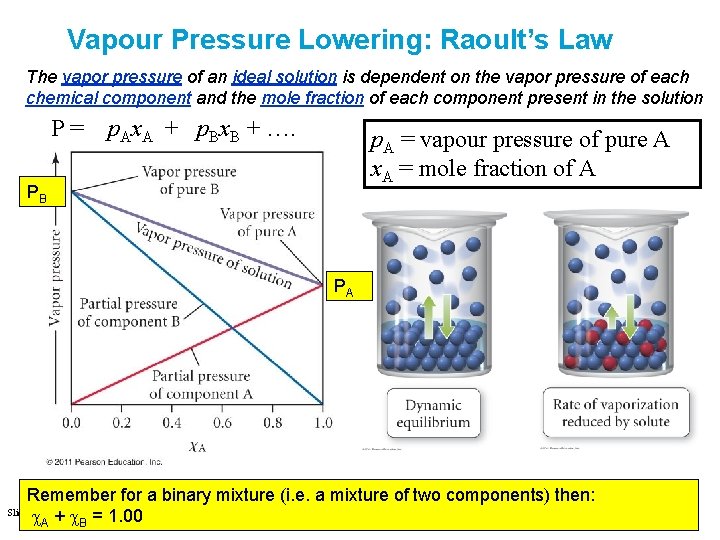
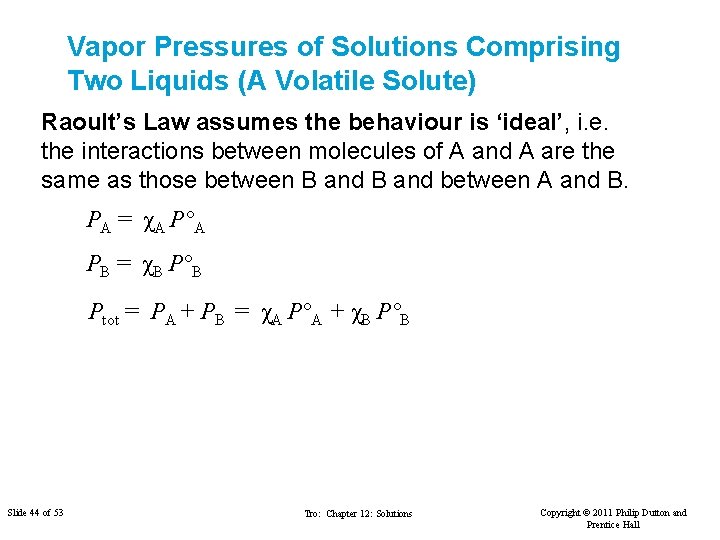
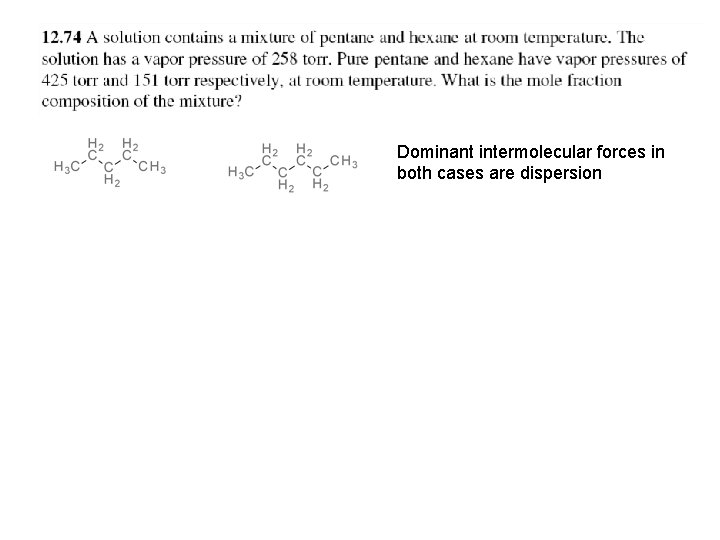
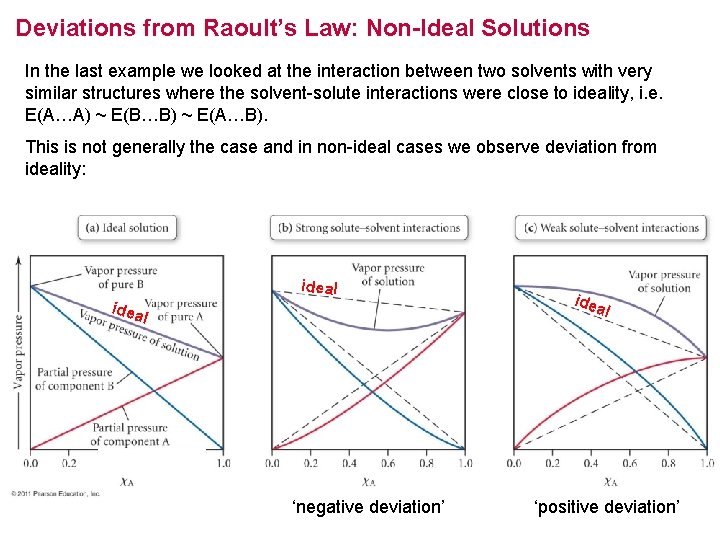
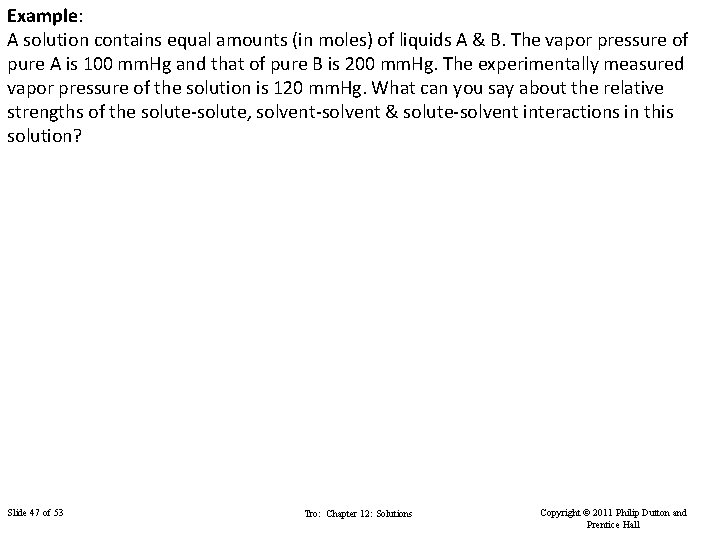
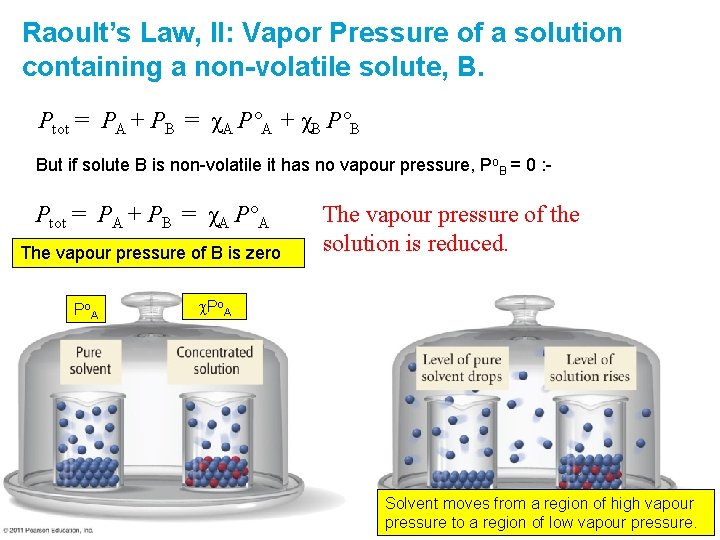
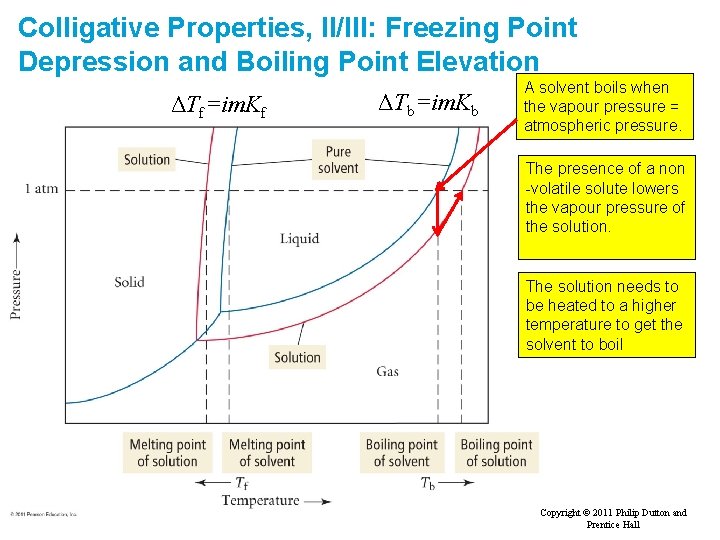
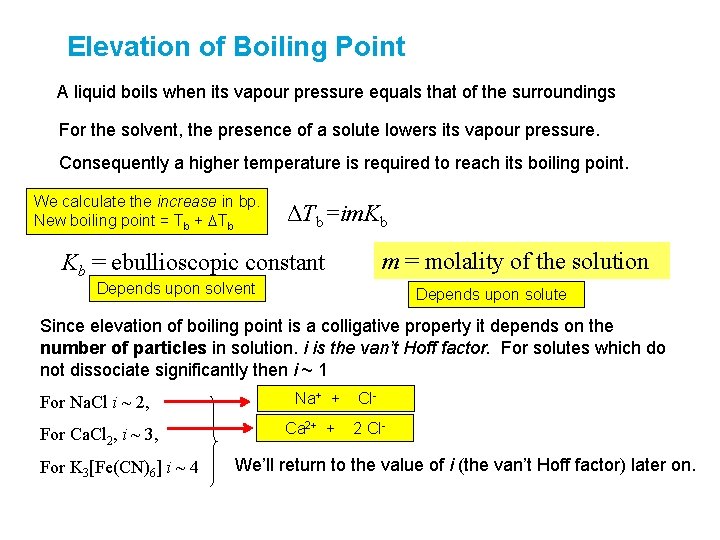
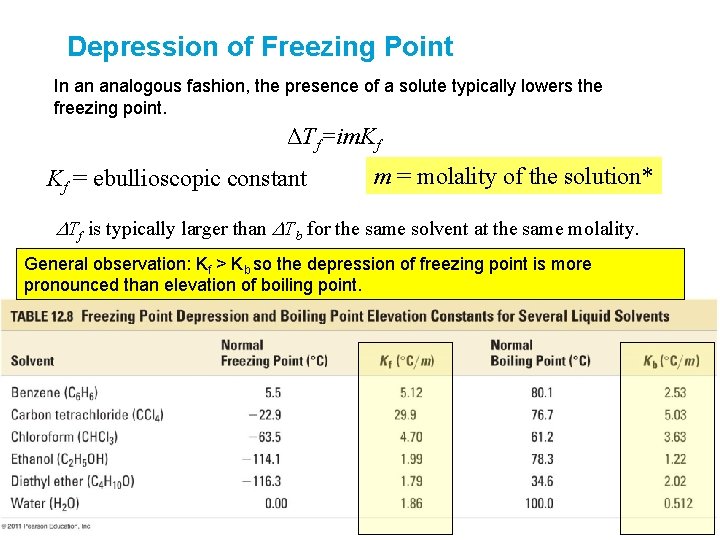
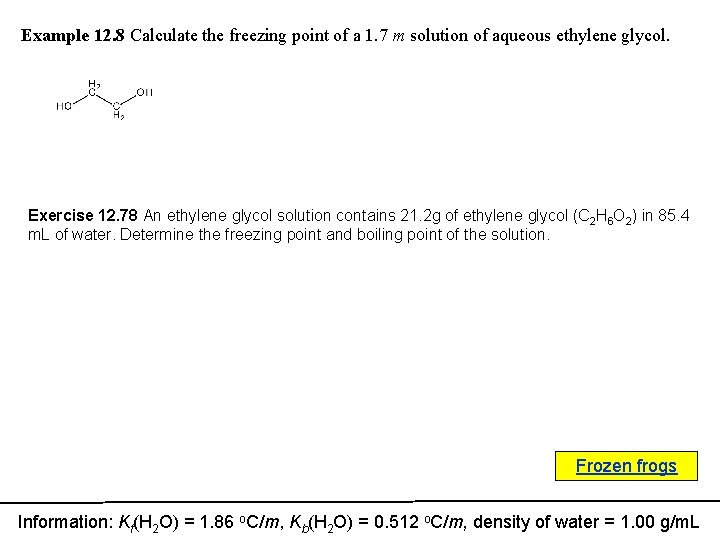
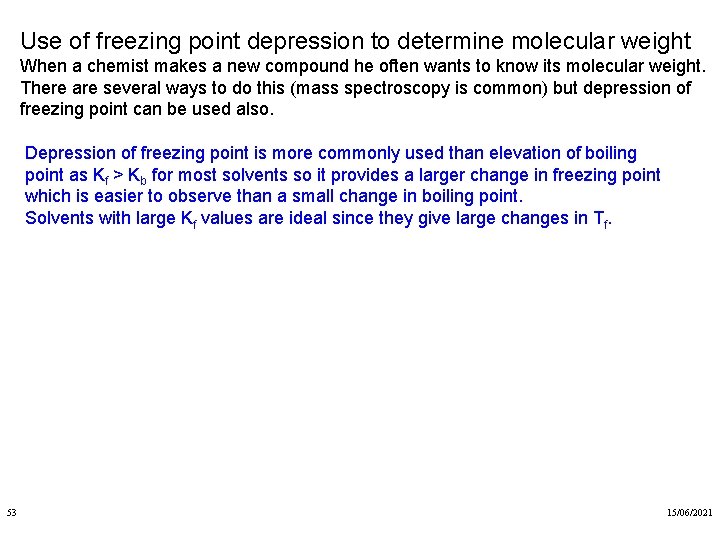
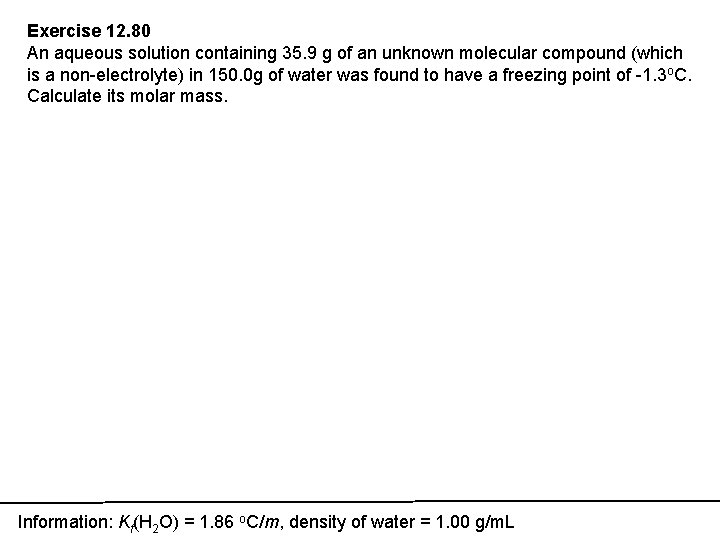
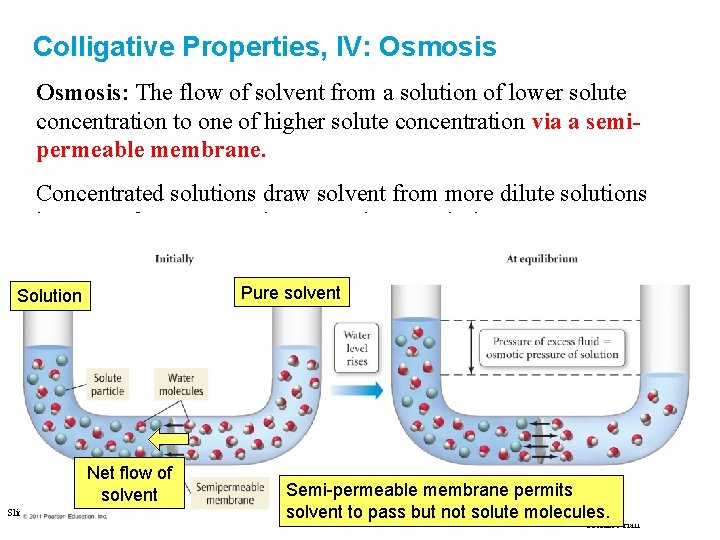
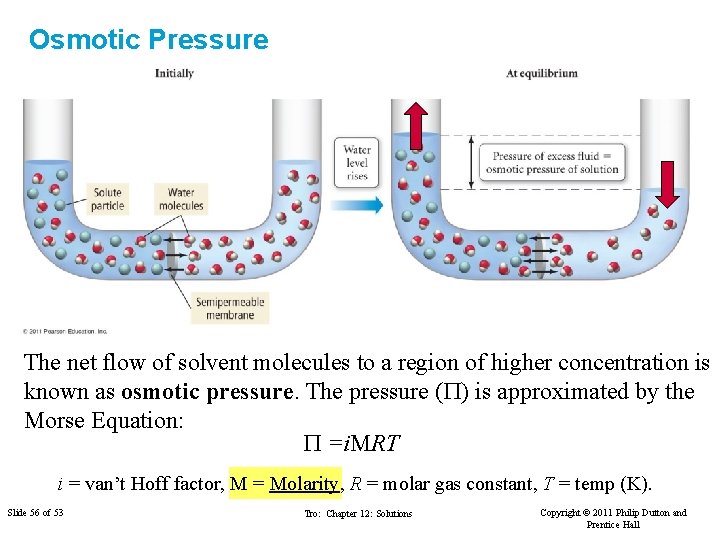
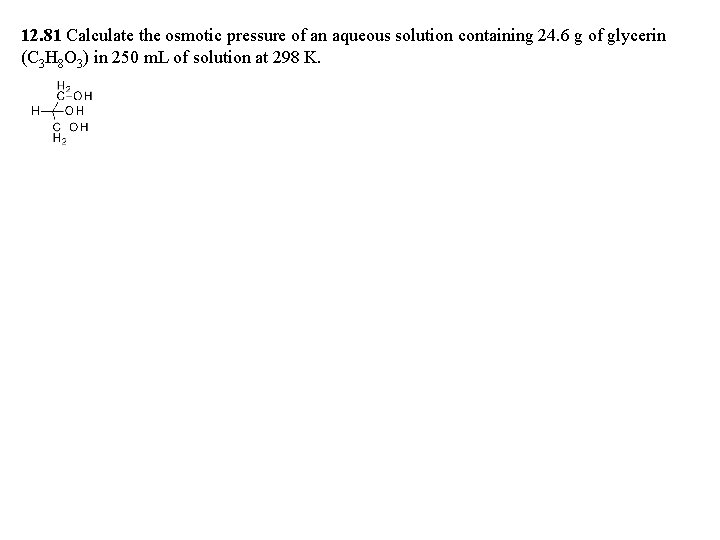
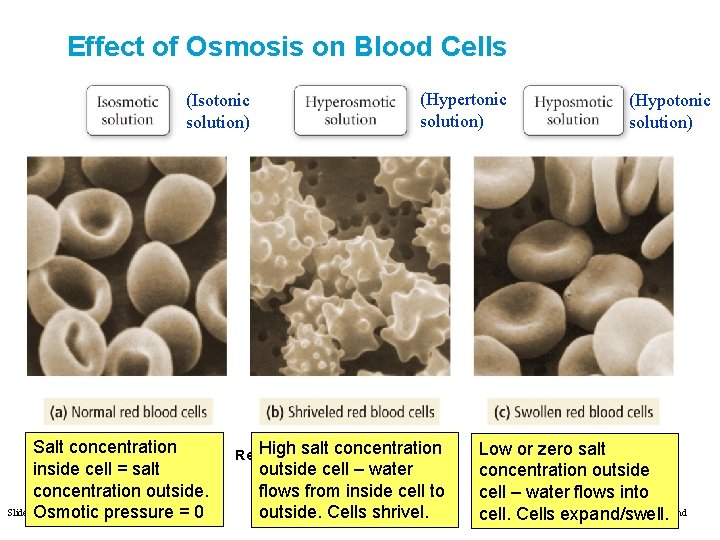
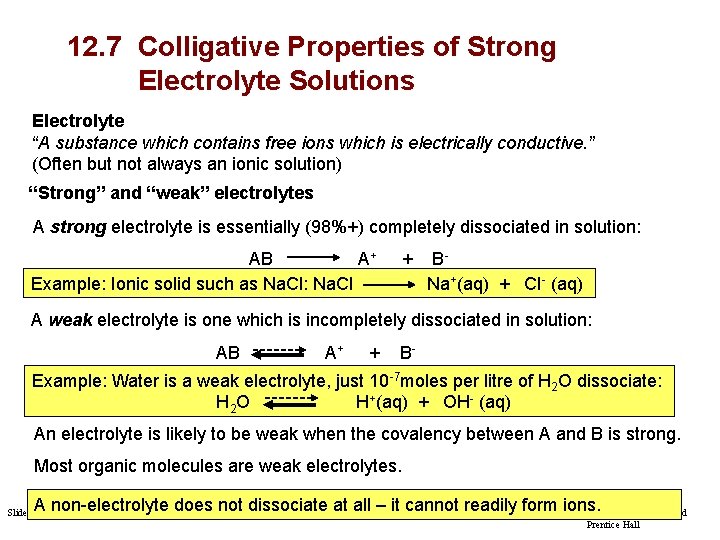
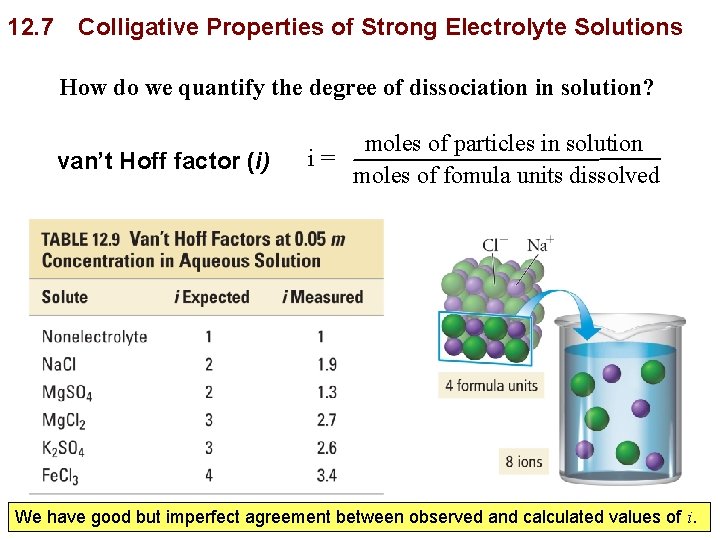
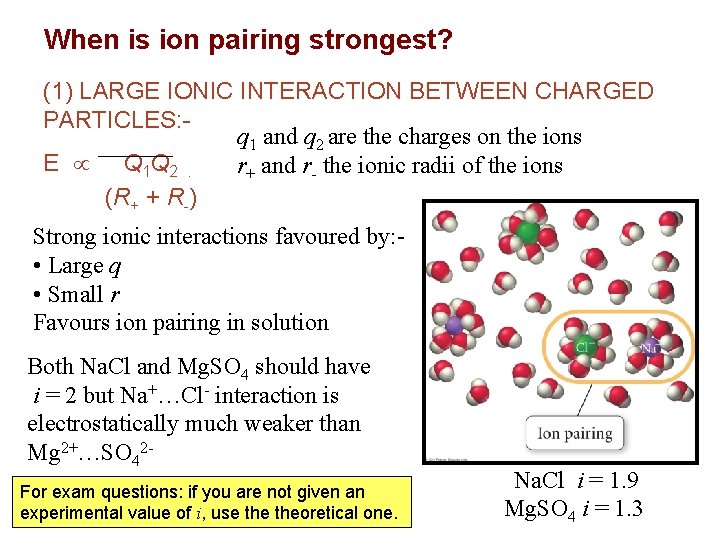
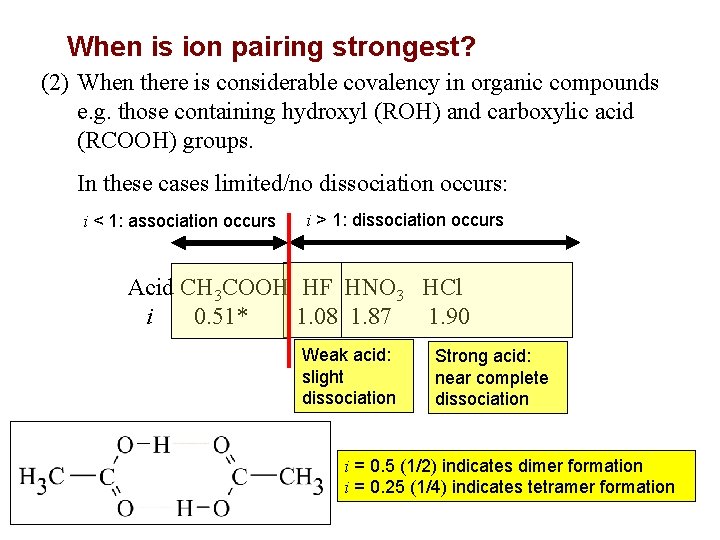
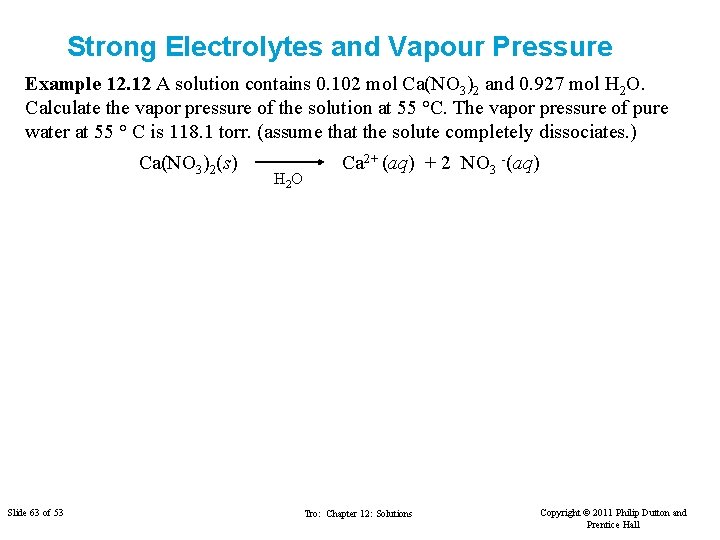
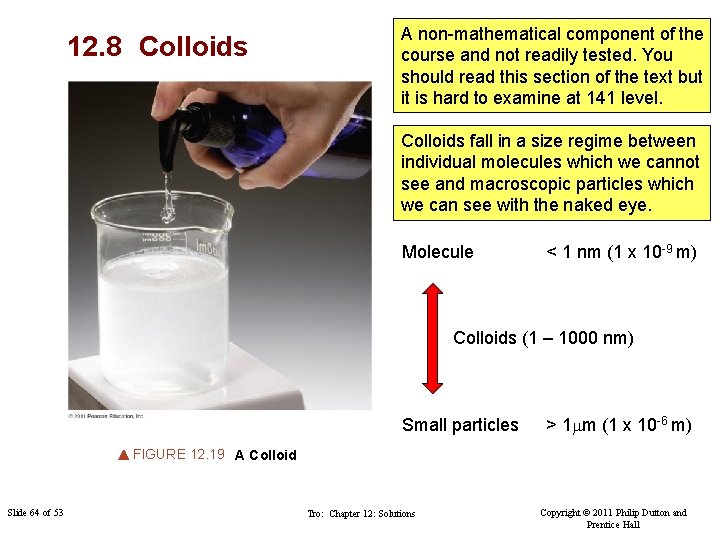
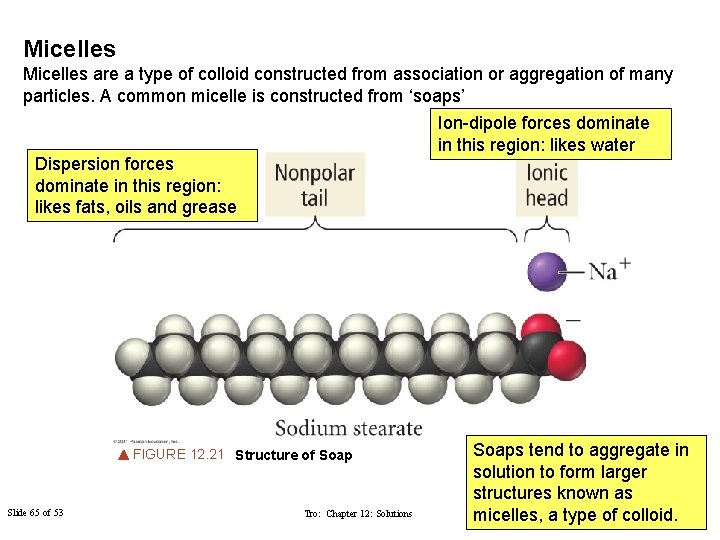
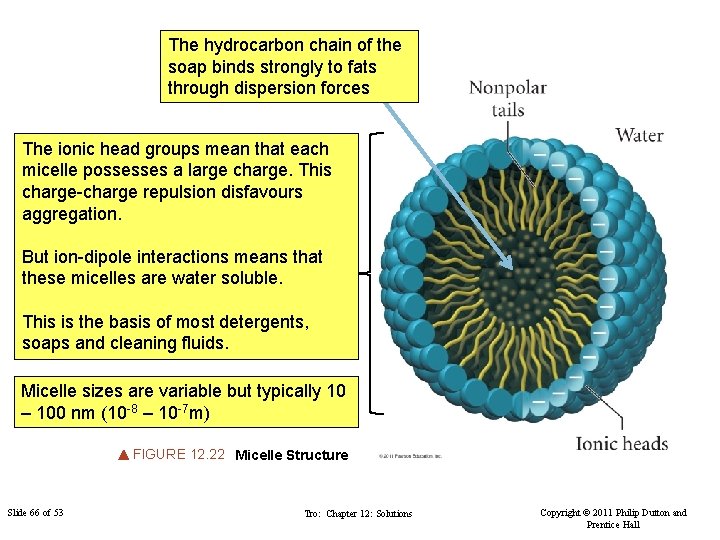
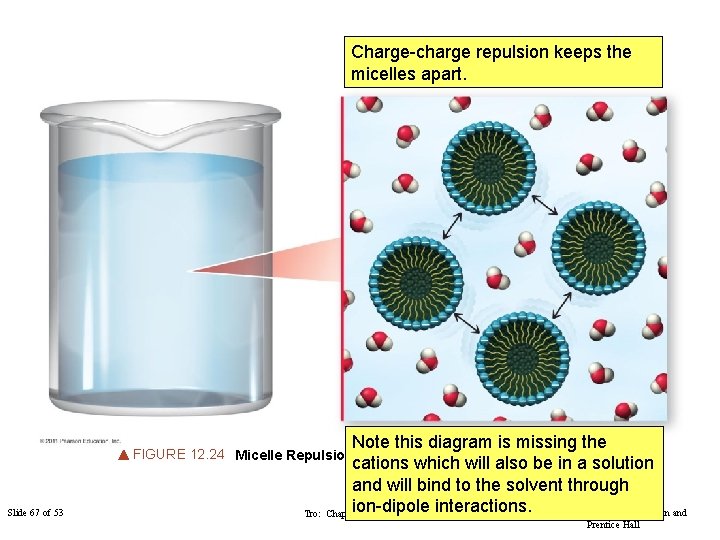
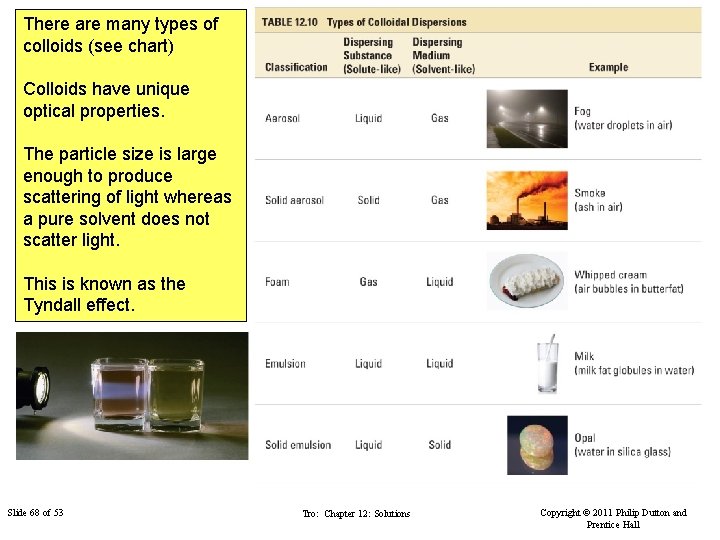
- Slides: 68
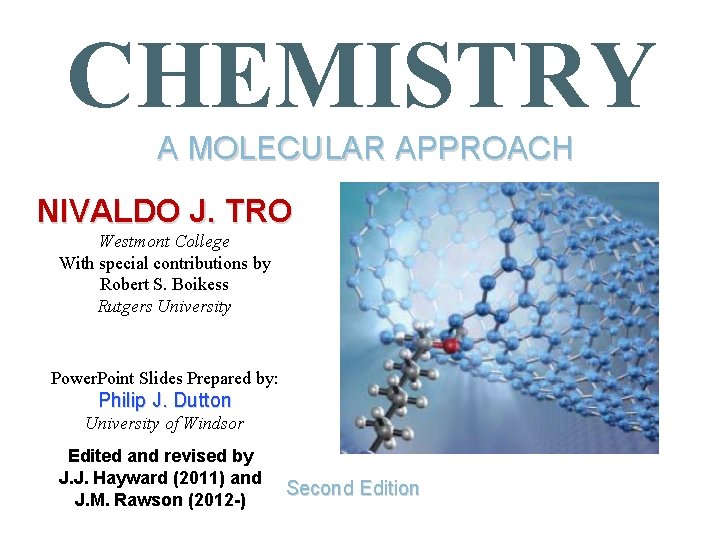
CHEMISTRY A MOLECULAR APPROACH NIVALDO J. TRO Westmont College With special contributions by Robert S. Boikess Rutgers University Power. Point Slides Prepared by: Philip J. Dutton University of Windsor Edited and revised by J. J. Hayward (2011) and J. M. Rawson (2012 -) Second Edition
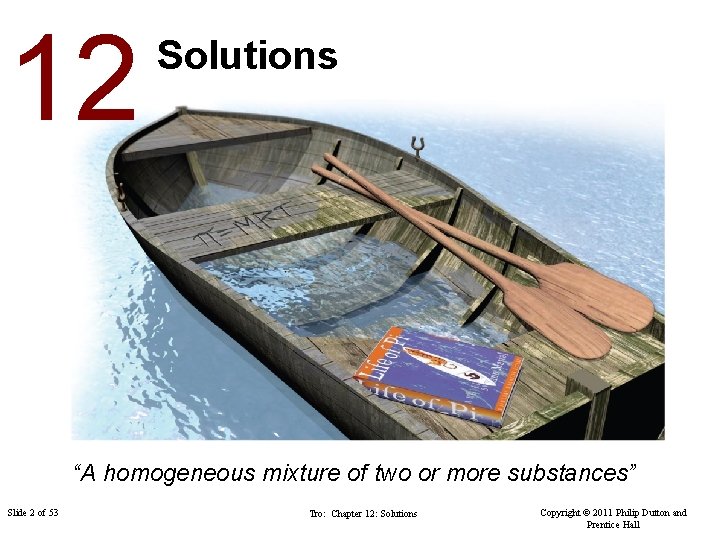
12 Solutions “A homogeneous mixture of two or more substances” Slide 2 of 53 Tro: Chapter 12: Solutions Copyright © 2011 Philip Dutton and Prentice Hall
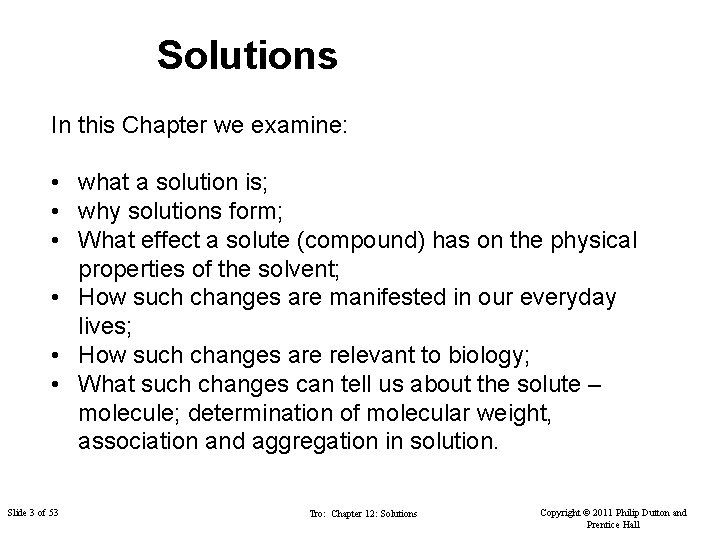
Solutions In this Chapter we examine: • what a solution is; • why solutions form; • What effect a solute (compound) has on the physical properties of the solvent; • How such changes are manifested in our everyday lives; • How such changes are relevant to biology; • What such changes can tell us about the solute – molecule; determination of molecular weight, association and aggregation in solution. Slide 3 of 53 Tro: Chapter 12: Solutions Copyright © 2011 Philip Dutton and Prentice Hall
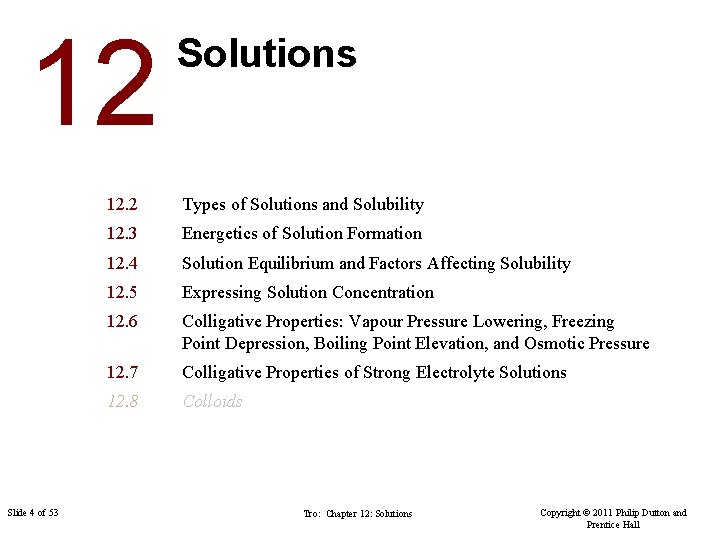
12 Slide 4 of 53 Solutions 12. 2 Types of Solutions and Solubility 12. 3 Energetics of Solution Formation 12. 4 Solution Equilibrium and Factors Affecting Solubility 12. 5 Expressing Solution Concentration 12. 6 Colligative Properties: Vapour Pressure Lowering, Freezing Point Depression, Boiling Point Elevation, and Osmotic Pressure 12. 7 Colligative Properties of Strong Electrolyte Solutions 12. 8 Colloids Tro: Chapter 12: Solutions Copyright © 2011 Philip Dutton and Prentice Hall
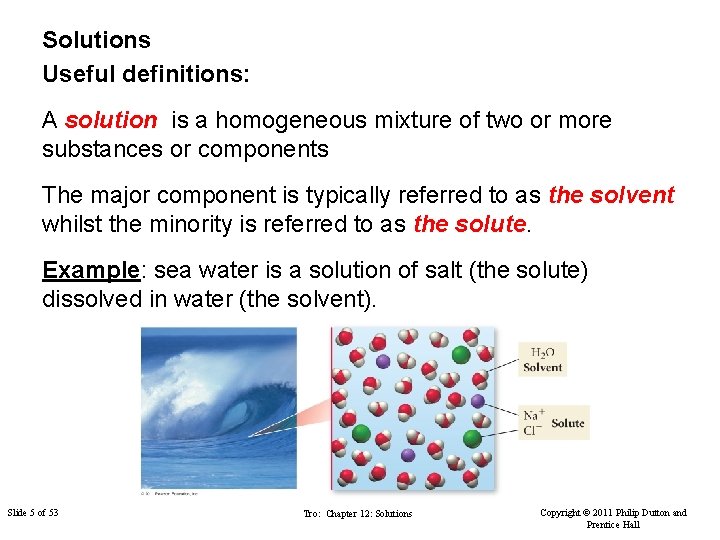
Solutions Useful definitions: A solution is a homogeneous mixture of two or more substances or components The major component is typically referred to as the solvent whilst the minority is referred to as the solute. Example: sea water is a solution of salt (the solute) dissolved in water (the solvent). Slide 5 of 53 Tro: Chapter 12: Solutions Copyright © 2011 Philip Dutton and Prentice Hall
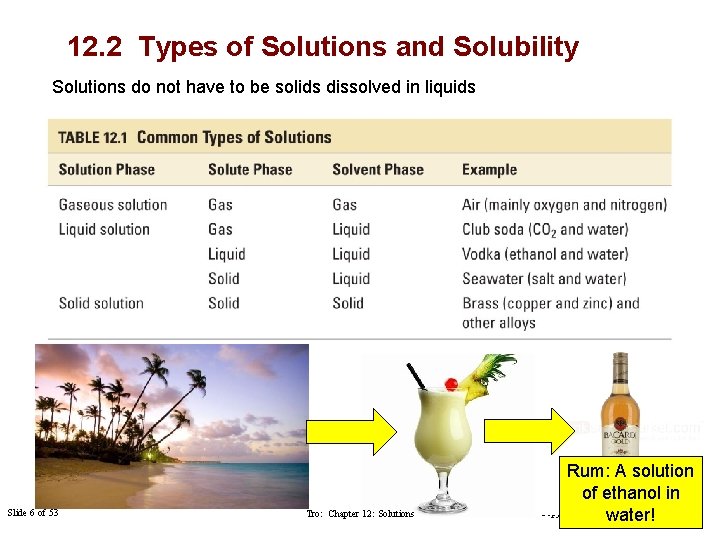
12. 2 Types of Solutions and Solubility Solutions do not have to be solids dissolved in liquids Slide 6 of 53 Tro: Chapter 12: Solutions Rum: A solution of ethanol in Copyright © 2011 Philip Dutton and water! Prentice Hall
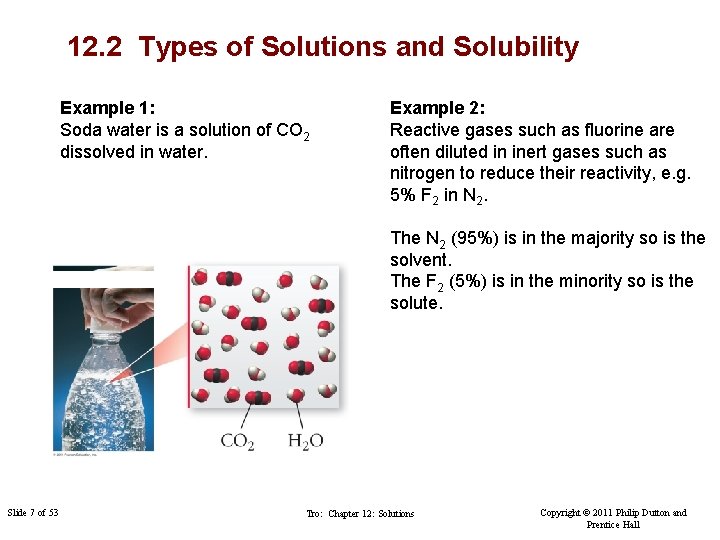
12. 2 Types of Solutions and Solubility Example 1: Soda water is a solution of CO 2 dissolved in water. Example 2: Reactive gases such as fluorine are often diluted in inert gases such as nitrogen to reduce their reactivity, e. g. 5% F 2 in N 2. The N 2 (95%) is in the majority so is the solvent. The F 2 (5%) is in the minority so is the solute. Slide 7 of 53 Tro: Chapter 12: Solutions Copyright © 2011 Philip Dutton and Prentice Hall
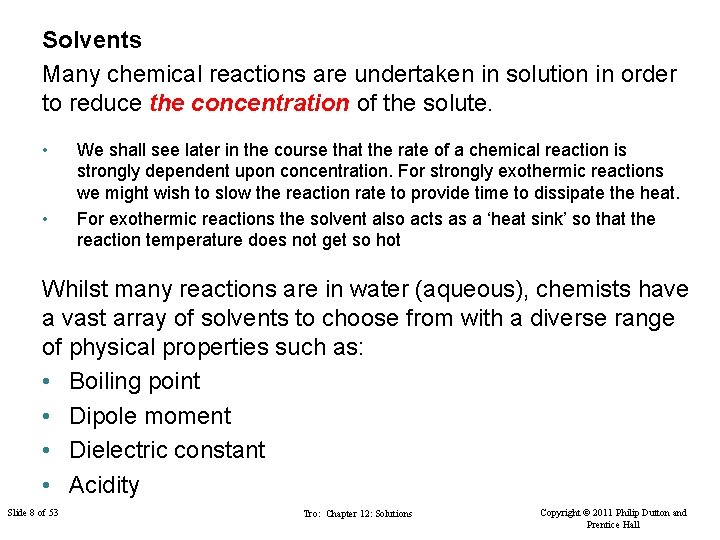
Solvents Many chemical reactions are undertaken in solution in order to reduce the concentration of the solute. • • We shall see later in the course that the rate of a chemical reaction is strongly dependent upon concentration. For strongly exothermic reactions we might wish to slow the reaction rate to provide time to dissipate the heat. For exothermic reactions the solvent also acts as a ‘heat sink’ so that the reaction temperature does not get so hot Whilst many reactions are in water (aqueous), chemists have a vast array of solvents to choose from with a diverse range of physical properties such as: • Boiling point • Dipole moment • Dielectric constant • Acidity Slide 8 of 53 Tro: Chapter 12: Solutions Copyright © 2011 Philip Dutton and Prentice Hall
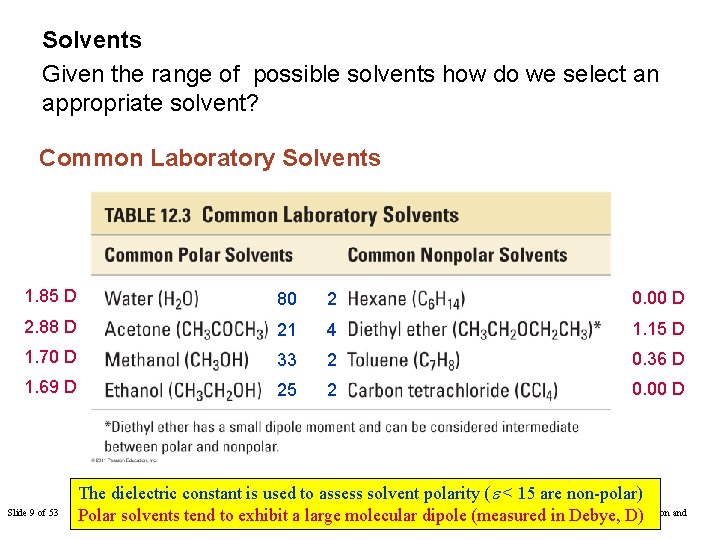
Solvents Given the range of possible solvents how do we select an appropriate solvent? Common Laboratory Solvents 1. 85 D 80 2 0. 00 D 2. 88 D 21 4 1. 15 D 1. 70 D 33 2 0. 36 D 1. 69 D 25 2 0. 00 D Slide 9 of 53 The dielectric constant is used to assess solvent polarity (e < 15 are non-polar) Copyright © 2011 Philip Chapter 12: Solutions dipole (measured Polar solvents tend to exhibit a Tro: large molecular in Debye, D)Dutton and Prentice Hall
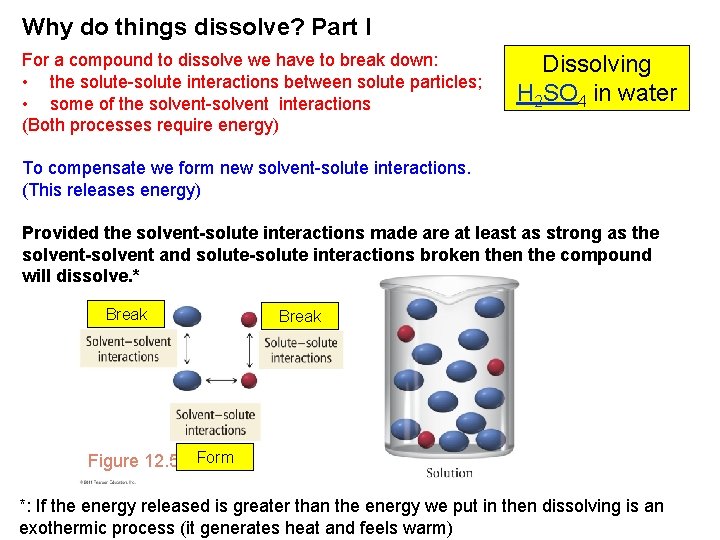
Why do things dissolve? Part I For a compound to dissolve we have to break down: • the solute-solute interactions between solute particles; • some of the solvent-solvent interactions (Both processes require energy) Dissolving H 2 SO 4 in water To compensate we form new solvent-solute interactions. (This releases energy) Provided the solvent-solute interactions made are at least as strong as the solvent-solvent and solute-solute interactions broken the compound will dissolve. * Break Figure. FIGURE 12. 5 Form *: If the energy released is greater than the energy we put in then dissolving is an exothermic process (it generates heat and feels warm)
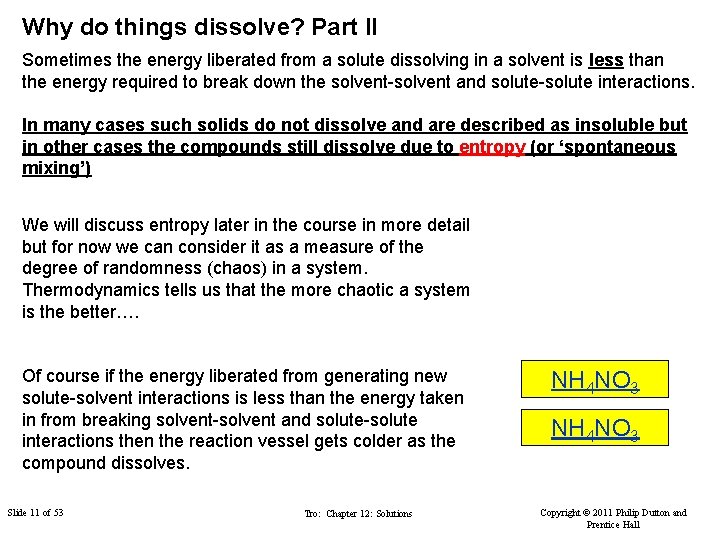
Why do things dissolve? Part II Sometimes the energy liberated from a solute dissolving in a solvent is less than the energy required to break down the solvent-solvent and solute-solute interactions. In many cases such solids do not dissolve and are described as insoluble but in other cases the compounds still dissolve due to entropy (or ‘spontaneous mixing’) We will discuss entropy later in the course in more detail but for now we can consider it as a measure of the degree of randomness (chaos) in a system. Thermodynamics tells us that the more chaotic a system is the better…. Of course if the energy liberated from generating new solute-solvent interactions is less than the energy taken in from breaking solvent-solvent and solute-solute interactions then the reaction vessel gets colder as the FIGURE compound dissolves. Slide 11 of 53 Tro: Chapter 12: Solutions NH 4 NO 3 Copyright © 2011 Philip Dutton and Prentice Hall
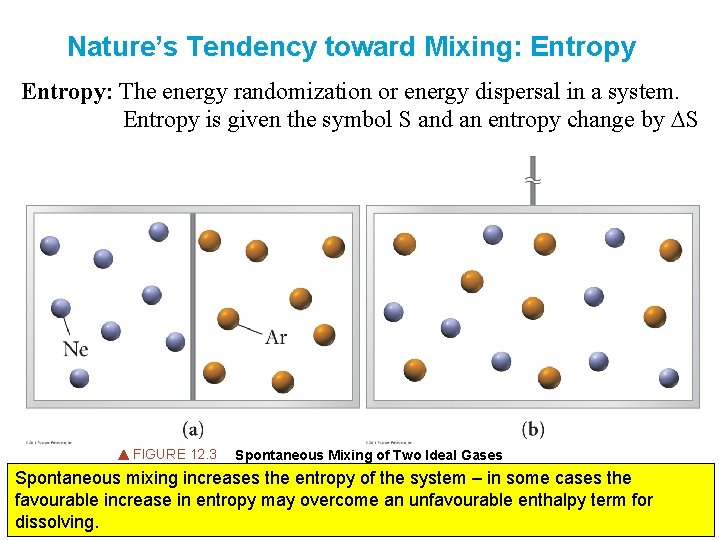
Nature’s Tendency toward Mixing: Entropy: The energy randomization or energy dispersal in a system. Entropy is given the symbol S and an entropy change by DS FIGURE 12. 3 Spontaneous Mixing of Two Ideal Gases Spontaneous mixing increases the entropy of the system – in some cases the favourable increase in entropy may overcome an unfavourable enthalpy term for dissolving.
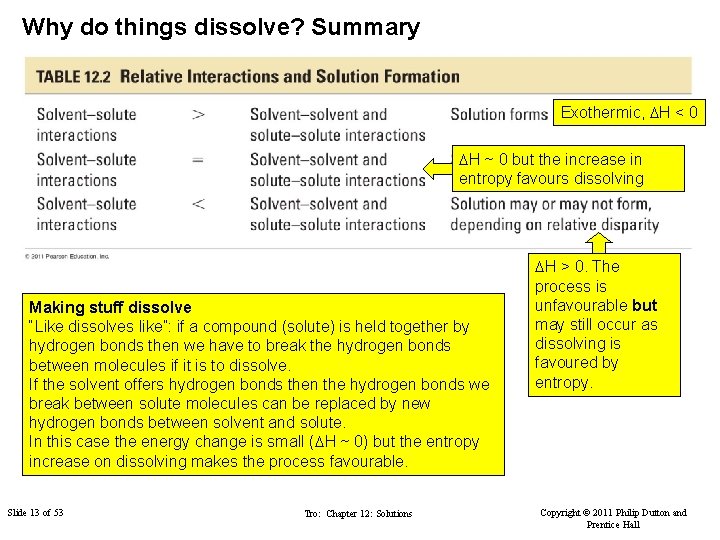
Why do things dissolve? Summary Exothermic, DH < 0 DH ~ 0 but the increase in entropy favours dissolving Making stuff dissolve “Like dissolves like”: if a compound (solute) is held together by hydrogen bonds then we have to break the hydrogen bonds between molecules if it is to dissolve. If the solvent offers hydrogen bonds then the hydrogen bonds we break between solute molecules can be replaced by new hydrogen bonds between solvent and solute. In this case the energy change is small (DH ~ 0) but the entropy FIGURE increase on dissolving makes the process favourable. Slide 13 of 53 Tro: Chapter 12: Solutions DH > 0. The process is unfavourable but may still occur as dissolving is favoured by entropy. Copyright © 2011 Philip Dutton and Prentice Hall
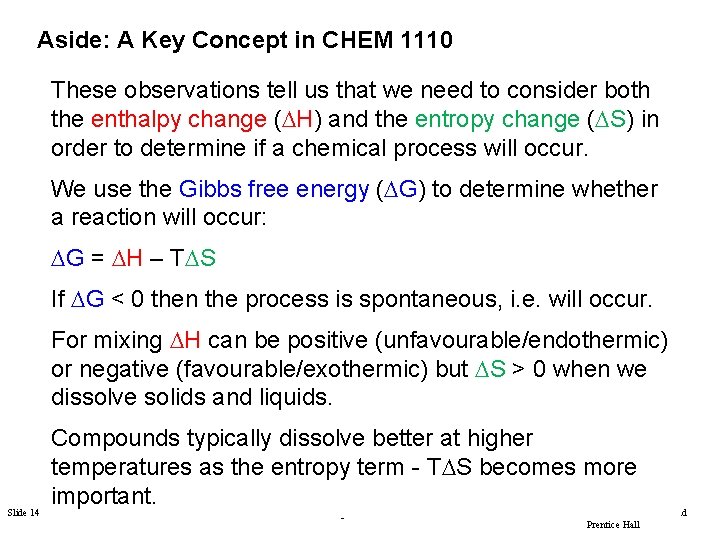
Aside: A Key Concept in CHEM 1110 These observations tell us that we need to consider both the enthalpy change (DH) and the entropy change (DS) in order to determine if a chemical process will occur. We use the Gibbs free energy (DG) to determine whether a reaction will occur: DG = DH – TDS If DG < 0 then the process is spontaneous, i. e. will occur. For mixing DH can be positive (unfavourable/endothermic) or negative (favourable/exothermic) but DS > 0 when we dissolve solids and liquids. Compounds typically dissolve better at higher FIGURE temperatures as the entropy term - TDS becomes more important. Slide 14 of 53 Tro: Chapter 12: Solutions Copyright © 2011 Philip Dutton and Prentice Hall
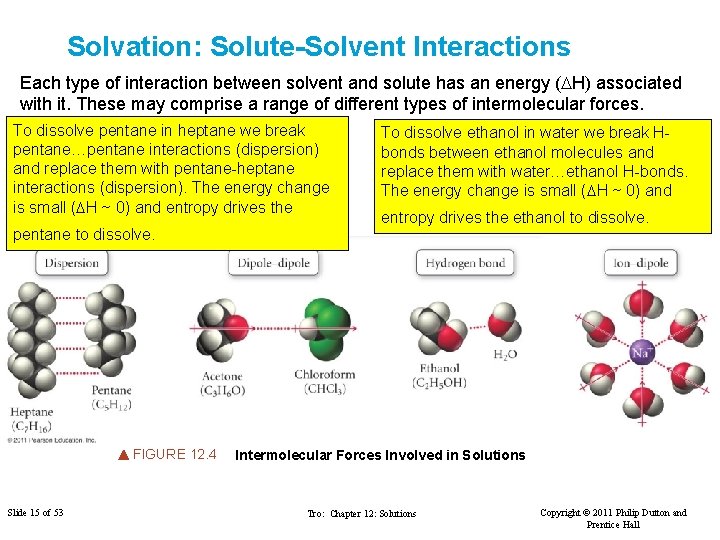
Solvation: Solute-Solvent Interactions Each type of interaction between solvent and solute has an energy (DH) associated with it. These may comprise a range of different types of intermolecular forces. To dissolve pentane in heptane we break pentane…pentane interactions (dispersion) and replace them with pentane-heptane interactions (dispersion). The energy change is small (DH ~ 0) and entropy drives the pentane to dissolve. FIGURE 12. 4 Slide 15 of 53 To dissolve ethanol in water we break Hbonds between ethanol molecules and replace them with water…ethanol H-bonds. The energy change is small (DH ~ 0) and entropy drives the ethanol to dissolve. Intermolecular Forces Involved in Solutions Tro: Chapter 12: Solutions Copyright © 2011 Philip Dutton and Prentice Hall
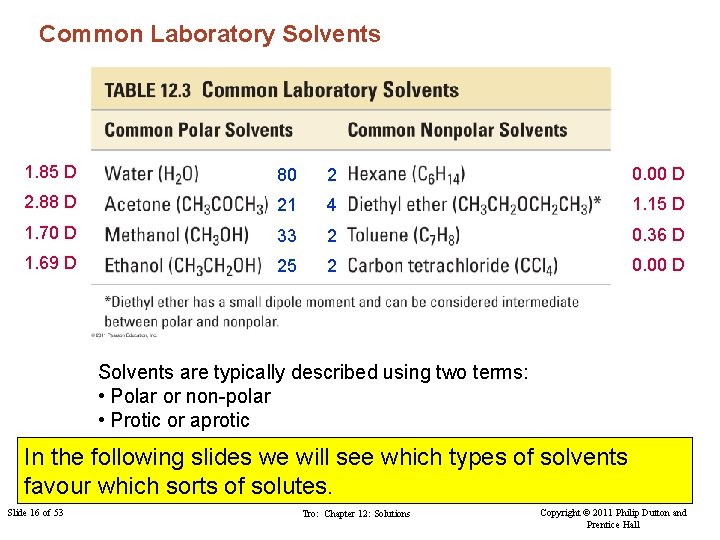
Common Laboratory Solvents 1. 85 D 80 2 0. 00 D 2. 88 D 21 4 1. 15 D 1. 70 D 33 2 0. 36 D 1. 69 D 25 2 0. 00 D Solvents are typically described using two terms: • Polar or non-polar • Protic or aprotic In the following slides we will see which types of solvents favour which sorts of solutes. Slide 16 of 53 Tro: Chapter 12: Solutions Copyright © 2011 Philip Dutton and Prentice Hall
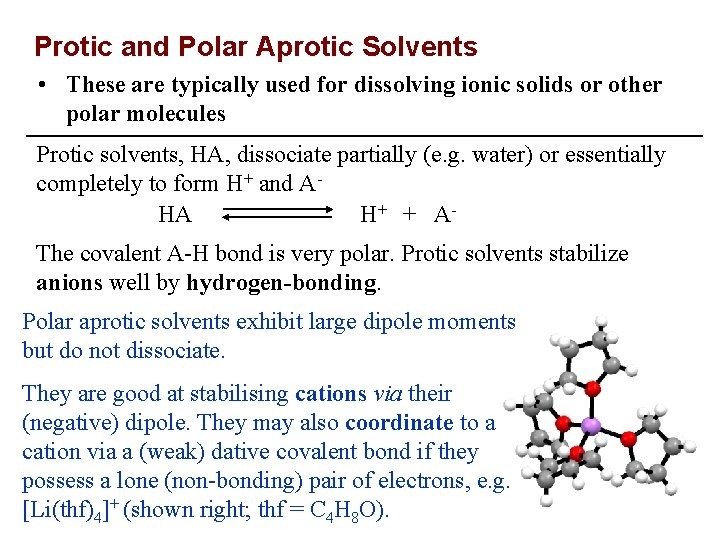
Protic and Polar Aprotic Solvents • These are typically used for dissolving ionic solids or other polar molecules Protic solvents, HA, dissociate partially (e. g. water) or essentially completely to form H+ and AHA H+ + A The covalent A-H bond is very polar. Protic solvents stabilize anions well by hydrogen-bonding. Polar aprotic solvents exhibit large dipole moments but do not dissociate. They are good at stabilising cations via their (negative) dipole. They may also coordinate to a cation via a (weak) dative covalent bond if they possess a lone (non-bonding) pair of electrons, e. g. [Li(thf)4]+ (shown right; thf = C 4 H 8 O).
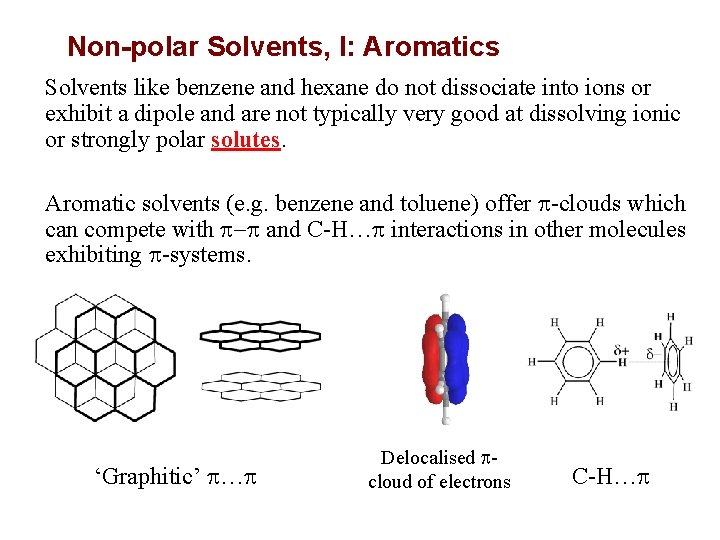
Non-polar Solvents, I: Aromatics Solvents like benzene and hexane do not dissociate into ions or exhibit a dipole and are not typically very good at dissolving ionic or strongly polar solutes. Aromatic solvents (e. g. benzene and toluene) offer p-clouds which can compete with p-p and C-H…p interactions in other molecules exhibiting p-systems. ‘Graphitic’ p…p Delocalised pcloud of electrons C-H…p
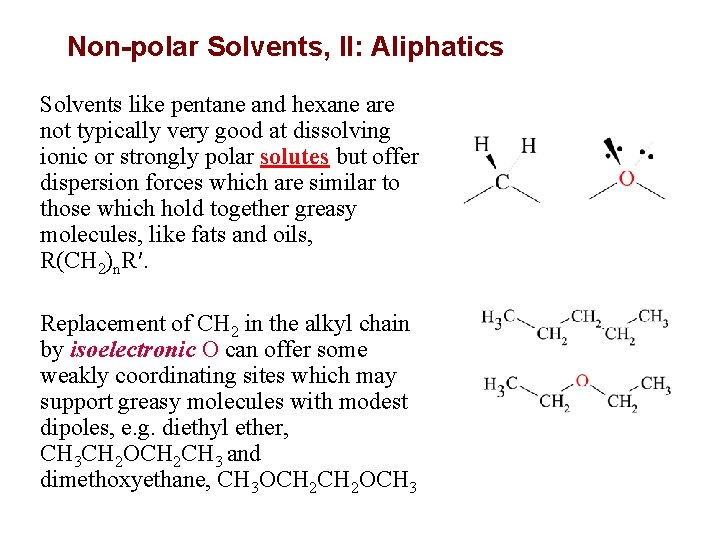
Non-polar Solvents, II: Aliphatics Solvents like pentane and hexane are not typically very good at dissolving ionic or strongly polar solutes but offer dispersion forces which are similar to those which hold together greasy molecules, like fats and oils, R(CH 2)n. R′. Replacement of CH 2 in the alkyl chain by isoelectronic O can offer some weakly coordinating sites which may support greasy molecules with modest dipoles, e. g. diethyl ether, CH 3 CH 2 OCH 2 CH 3 and dimethoxyethane, CH 3 OCH 2 OCH 3
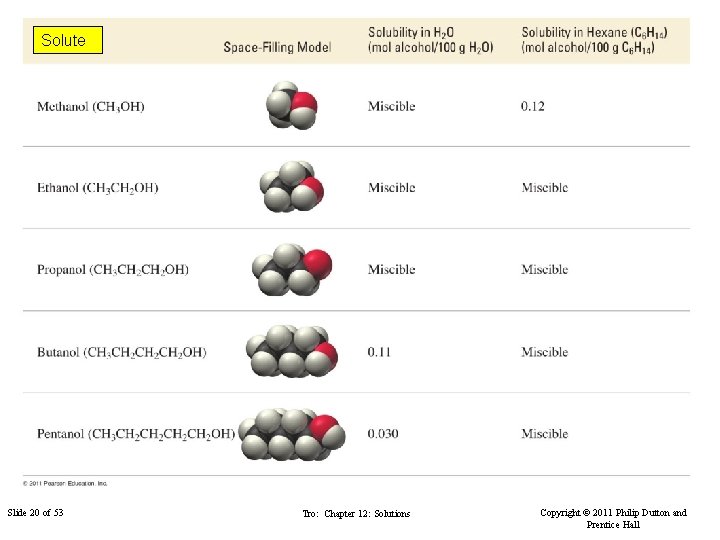
Solute Slide 20 of 53 Tro: Chapter 12: Solutions Copyright © 2011 Philip Dutton and Prentice Hall
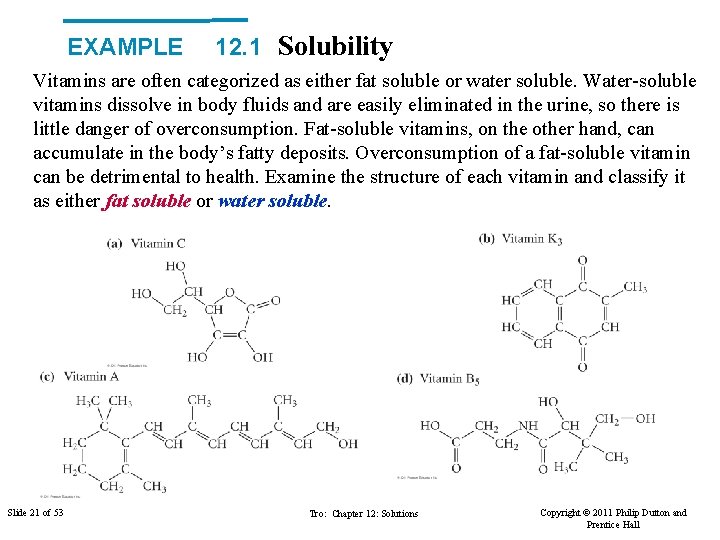
EXAMPLE 12. 1 Solubility Vitamins are often categorized as either fat soluble or water soluble. Water-soluble vitamins dissolve in body fluids and are easily eliminated in the urine, so there is little danger of overconsumption. Fat-soluble vitamins, on the other hand, can accumulate in the body’s fatty deposits. Overconsumption of a fat-soluble vitamin can be detrimental to health. Examine the structure of each vitamin and classify it as either fat soluble or water soluble. Slide 21 of 53 Tro: Chapter 12: Solutions Copyright © 2011 Philip Dutton and Prentice Hall
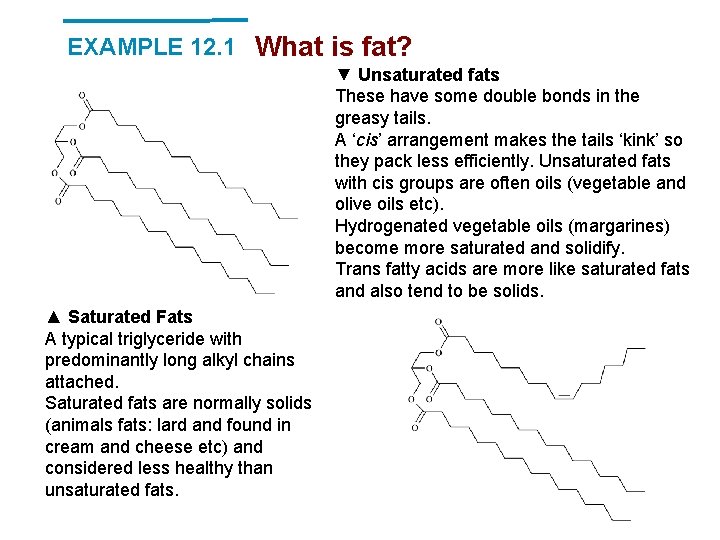
EXAMPLE 12. 1 What is fat? ▼ Unsaturated fats These have some double bonds in the greasy tails. A ‘cis’ arrangement makes the tails ‘kink’ so they pack less efficiently. Unsaturated fats with cis groups are often oils (vegetable and olive oils etc). Hydrogenated vegetable oils (margarines) become more saturated and solidify. Trans fatty acids are more like saturated fats and also tend to be solids. ▲ Saturated Fats A typical triglyceride with predominantly long alkyl chains attached. Saturated fats are normally solids (animals fats: lard and found in cream and cheese etc) and considered less healthy than unsaturated fats.
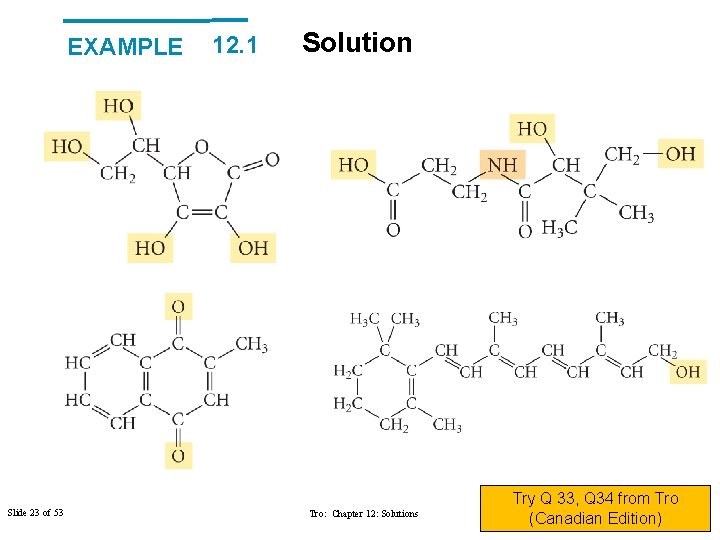
EXAMPLE Slide 23 of 53 12. 1 Solution Tro: Chapter 12: Solutions Try Q 33, Q 34 from Tro Copyright © 2011 Philip Dutton and (Canadian Edition) Prentice Hall
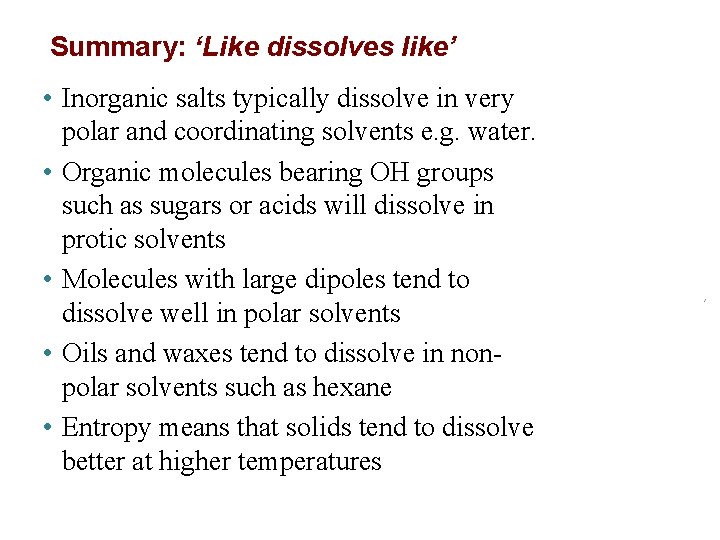
Summary: ‘Like dissolves like’ • Inorganic salts typically dissolve in very polar and coordinating solvents e. g. water. • Organic molecules bearing OH groups such as sugars or acids will dissolve in protic solvents • Molecules with large dipoles tend to dissolve well in polar solvents • Oils and waxes tend to dissolve in nonpolar solvents such as hexane • Entropy means that solids tend to dissolve better at higher temperatures
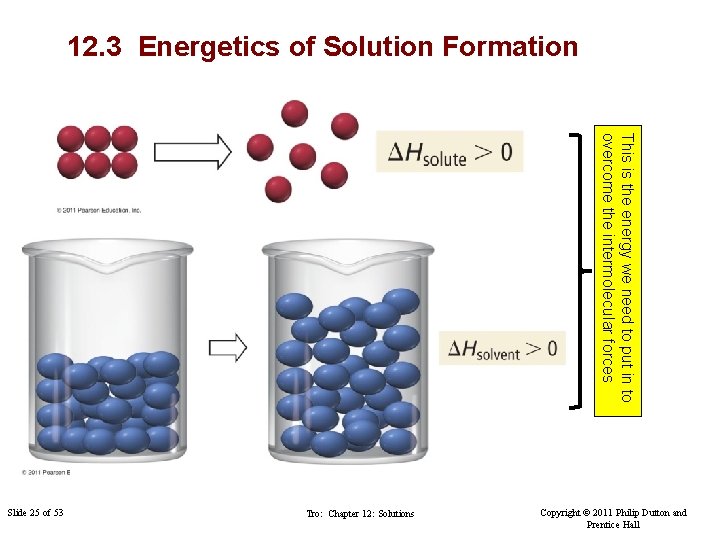
12. 3 Energetics of Solution Formation This is the energy we need to put in to overcome the intermolecular forces Slide 25 of 53 Tro: Chapter 12: Solutions Copyright © 2011 Philip Dutton and Prentice Hall
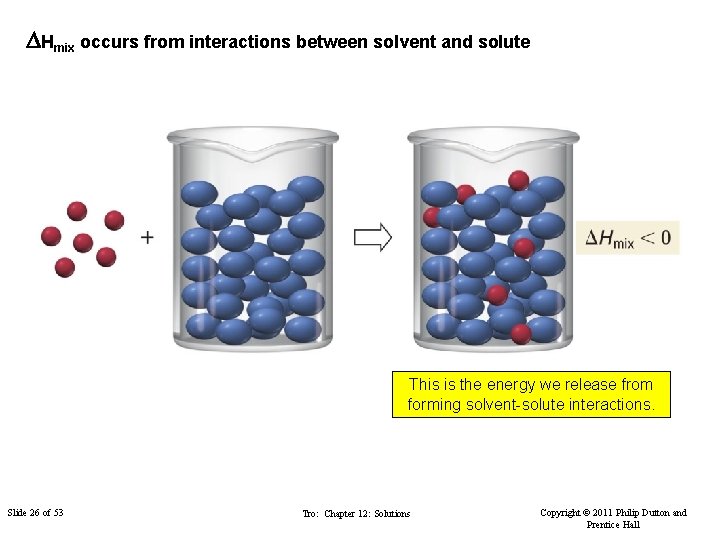
DHmix occurs from interactions between solvent and solute This is the energy we release from forming solvent-solute interactions. Slide 26 of 53 Tro: Chapter 12: Solutions Copyright © 2011 Philip Dutton and Prentice Hall
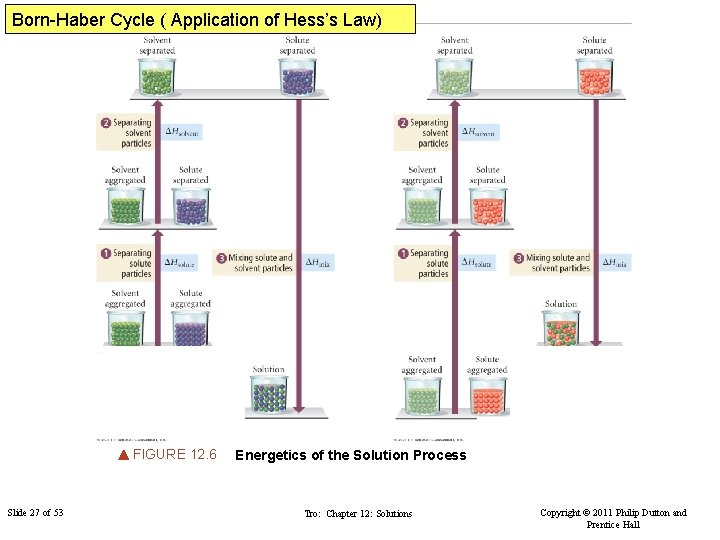
Born-Haber Cycle ( Application of Hess’s Law) FIGURE 12. 6 Slide 27 of 53 Energetics of the Solution Process Tro: Chapter 12: Solutions Copyright © 2011 Philip Dutton and Prentice Hall
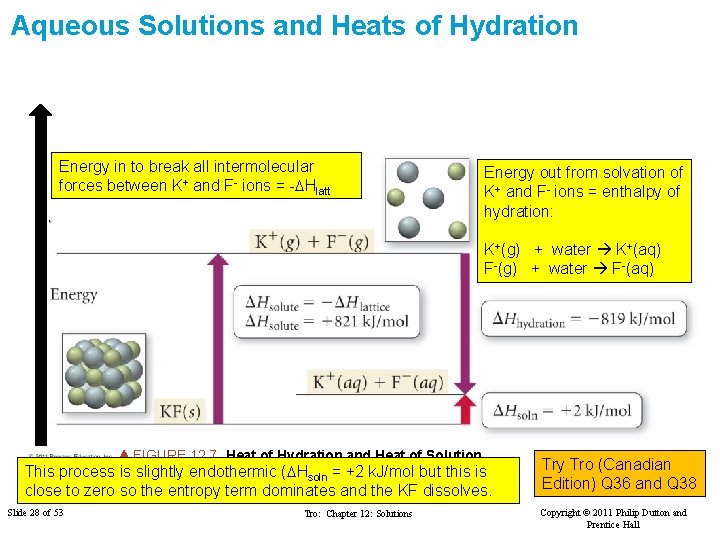
Aqueous Solutions and Heats of Hydration Energy in to break all intermolecular forces between K+ and F- ions = -DHlatt Energy out from solvation of K+ and F- ions = enthalpy of hydration: K+(g) + water K+(aq) F-(g) + water F-(aq) FIGURE 12. 7 Heat of Hydration and Heat of Solution This process is slightly endothermic (DHsoln = +2 k. J/mol but this is close to zero so the entropy term dominates and the KF dissolves. Slide 28 of 53 Tro: Chapter 12: Solutions Try Tro (Canadian Edition) Q 36 and Q 38 Copyright © 2011 Philip Dutton and Prentice Hall
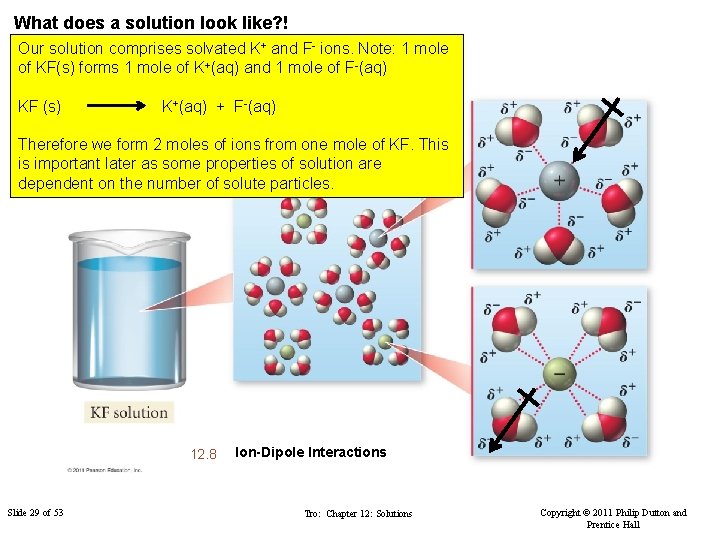
What does a solution look like? ! Our solution comprises solvated K+ and F- ions. Note: 1 mole of KF(s) forms 1 mole of K+(aq) and 1 mole of F-(aq) KF (s) K+(aq) + F-(aq) Therefore we form 2 moles of ions from one mole of KF. This is important later as some properties of solution are dependent on the number of solute particles. FIGURE 12. 8 Slide 29 of 53 Ion-Dipole Interactions Tro: Chapter 12: Solutions Copyright © 2011 Philip Dutton and Prentice Hall
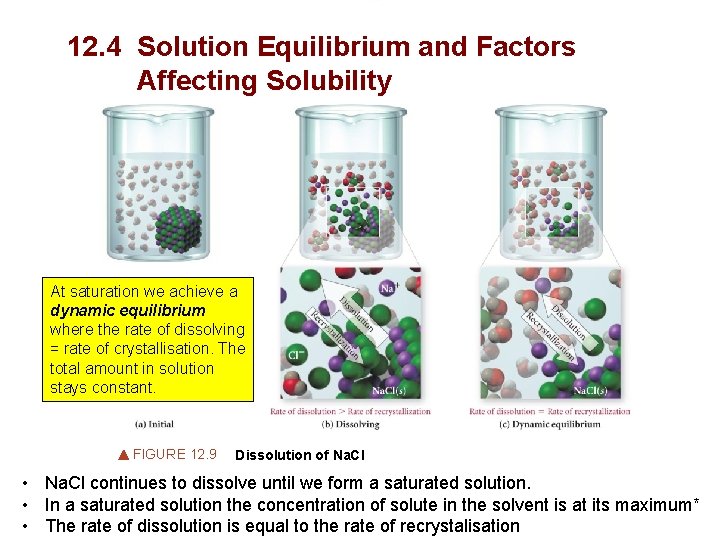
12. 4 Solution Equilibrium and Factors Affecting Solubility At saturation we achieve a dynamic equilibrium where the rate of dissolving = rate of crystallisation. The total amount in solution stays constant. FIGURE 12. 9 Dissolution of Na. Cl • Na. Cl continues to dissolve until we form a saturated solution. • In a saturated solution the concentration of solute in the solvent is at its maximum* • The rate of dissolution is equal to the rate of recrystalisation
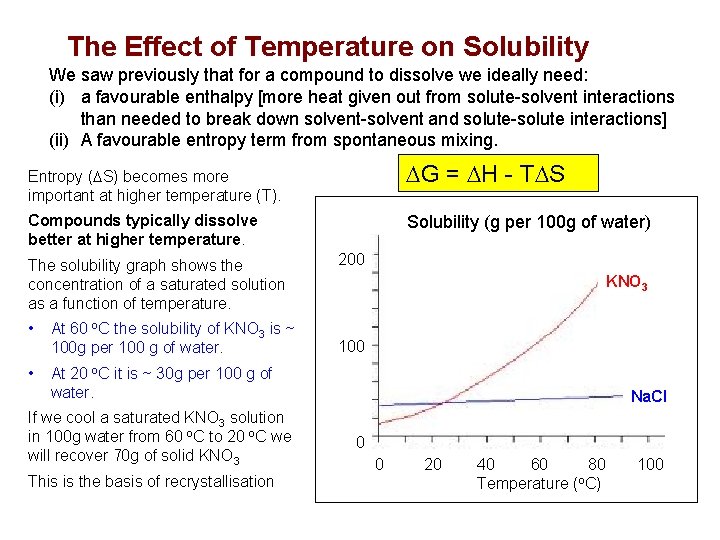
The Effect of Temperature on Solubility We saw previously that for a compound to dissolve we ideally need: (i) a favourable enthalpy [more heat given out from solute-solvent interactions than needed to break down solvent-solvent and solute-solute interactions] (ii) A favourable entropy term from spontaneous mixing. DG = DH - TDS Entropy (DS) becomes more important at higher temperature (T). Solubility (g per 100 g of water) Compounds typically dissolve better at higher temperature. The solubility graph shows the concentration of a saturated solution as a function of temperature. • At 60 o. C the solubility of KNO 3 is ~ 100 g per 100 g of water. • At 20 o. C it is ~ 30 g per 100 g of water. If we cool a saturated KNO 3 solution in 100 g water from 60 o. C to 20 o. C we will recover 70 g. FIGURE of solid KNO 3 This is the basis of recrystallisation 200 KNO 3 100 Na. Cl 0 0 20 40 60 80 Temperature (o. C) 100
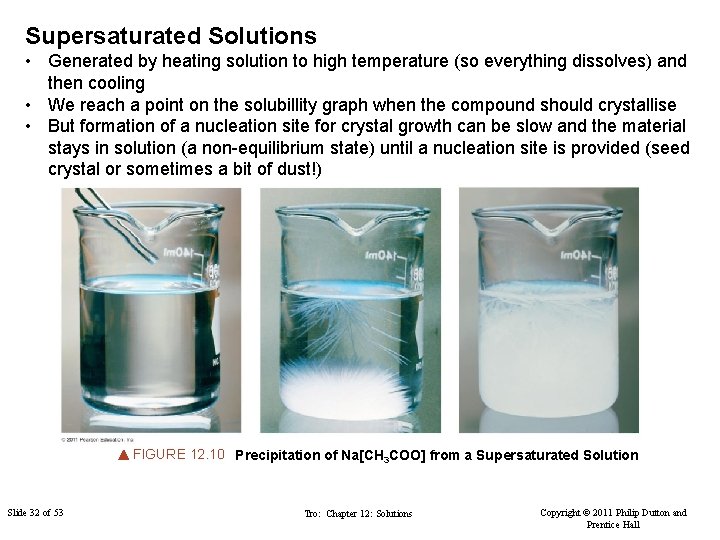
Supersaturated Solutions • Generated by heating solution to high temperature (so everything dissolves) and then cooling • We reach a point on the solubillity graph when the compound should crystallise • But formation of a nucleation site for crystal growth can be slow and the material stays in solution (a non-equilibrium state) until a nucleation site is provided (seed crystal or sometimes a bit of dust!) FIGURE 12. 10 Precipitation of Na[CH 3 COO] from a Supersaturated Solution Slide 32 of 53 Tro: Chapter 12: Solutions Copyright © 2011 Philip Dutton and Prentice Hall
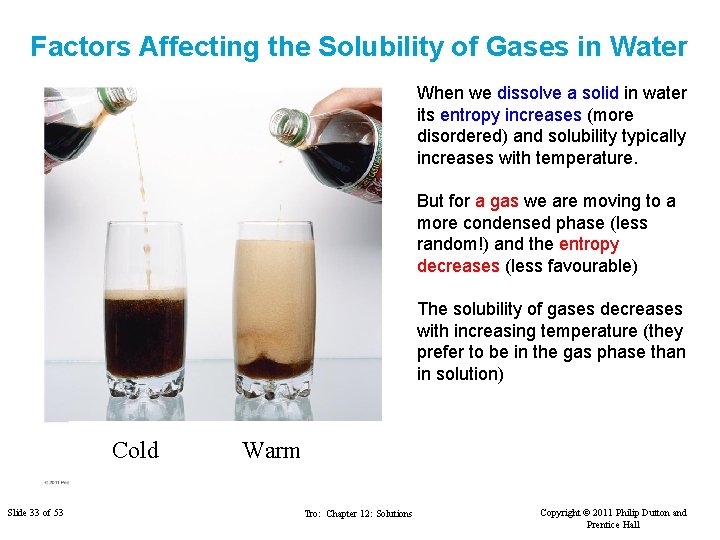
Factors Affecting the Solubility of Gases in Water When we dissolve a solid in water its entropy increases (more disordered) and solubility typically increases with temperature. But for a gas we are moving to a more condensed phase (less random!) and the entropy decreases (less favourable) The solubility of gases decreases with increasing temperature (they prefer to be in the gas phase than in solution) Cold Slide 33 of 53 Warm Tro: Chapter 12: Solutions Copyright © 2011 Philip Dutton and Prentice Hall
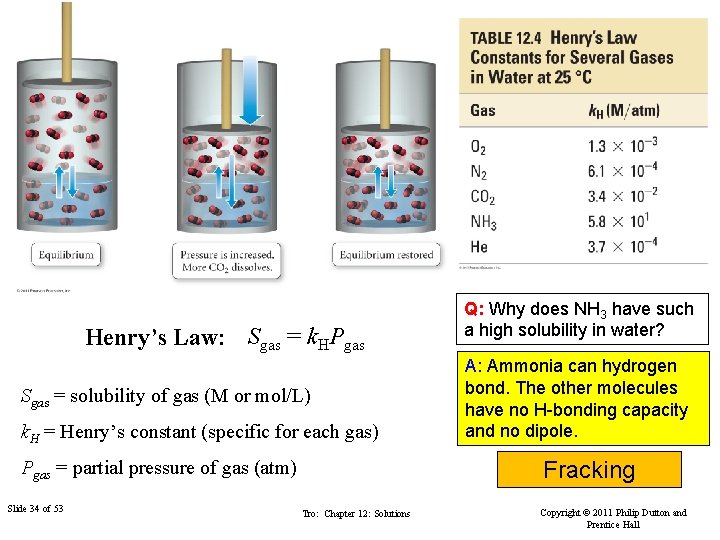
Henry’s Law: Sgas = k. HPgas Sgas = solubility of gas (M or mol/L) k. H = Henry’s constant (specific for each gas) Pgas = partial pressure of gas (atm) Slide 34 of 53 Q: Why does NH 3 have such a high solubility in water? A: Ammonia can hydrogen bond. The other molecules have no H-bonding capacity and no dipole. Fracking Tro: Chapter 12: Solutions Copyright © 2011 Philip Dutton and Prentice Hall
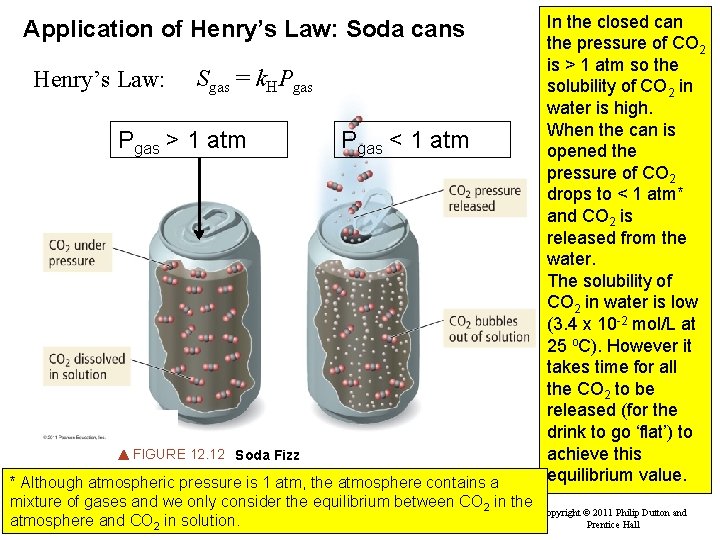
Application of Henry’s Law: Soda cans Henry’s Law: Sgas = k. HPgas > 1 atm Pgas < 1 atm FIGURE 12. 12 Soda Fizz * Although atmospheric pressure is 1 atm, the atmosphere contains a mixture of gases and we only consider the equilibrium between CO 2 in the Slide 35 of 53 Tro: Chapter 12: Solutions atmosphere and CO 2 in solution. In the closed can the pressure of CO 2 is > 1 atm so the solubility of CO 2 in water is high. When the can is opened the pressure of CO 2 drops to < 1 atm* and CO 2 is released from the water. The solubility of CO 2 in water is low (3. 4 x 10 -2 mol/L at 25 o. C). However it takes time for all the CO 2 to be released (for the drink to go ‘flat’) to achieve this equilibrium value. Copyright © 2011 Philip Dutton and Prentice Hall
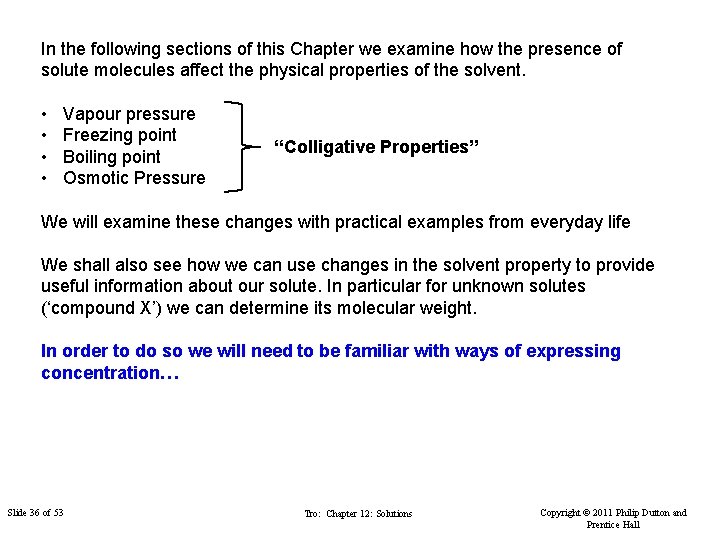
In the following sections of this Chapter we examine how the presence of solute molecules affect the physical properties of the solvent. • • Vapour pressure Freezing point Boiling point Osmotic Pressure “Colligative Properties” We will examine these changes with practical examples from everyday life We shall also see how we can use changes in the solvent property to provide useful information about our solute. In particular for unknown solutes (‘compound X’) we can determine its molecular weight. In order to do so we will need to be familiar with ways of expressing concentration… FIGURE Slide 36 of 53 Tro: Chapter 12: Solutions Copyright © 2011 Philip Dutton and Prentice Hall
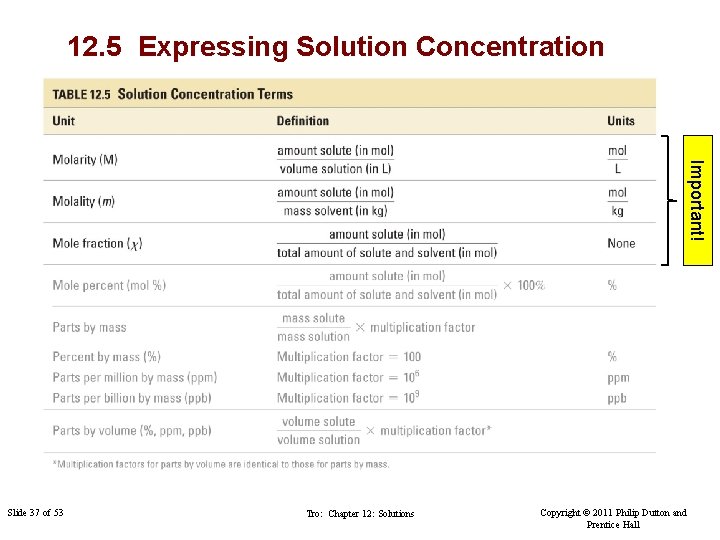
12. 5 Expressing Solution Concentration Important! Slide 37 of 53 Tro: Chapter 12: Solutions Copyright © 2011 Philip Dutton and Prentice Hall
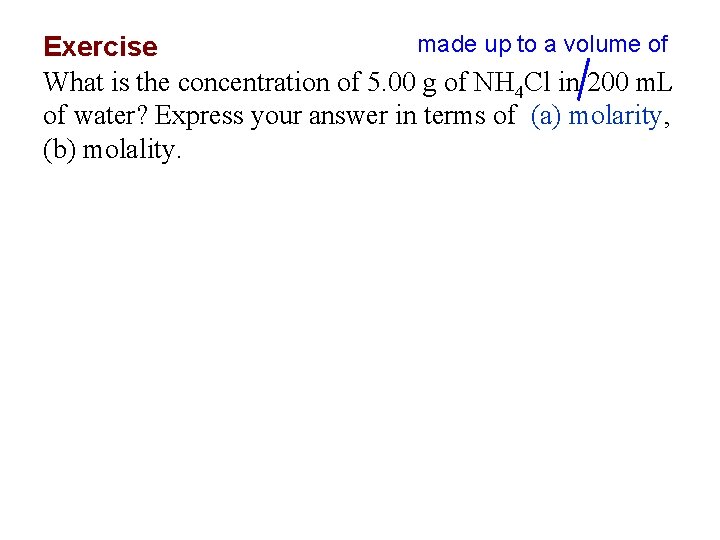
made up to a volume of Exercise What is the concentration of 5. 00 g of NH 4 Cl in 200 m. L of water? Express your answer in terms of (a) molarity, (b) molality.
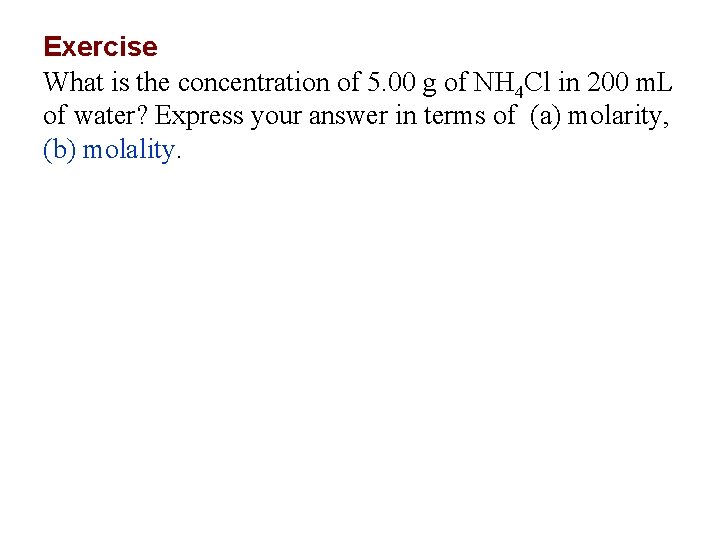
Exercise What is the concentration of 5. 00 g of NH 4 Cl in 200 m. L of water? Express your answer in terms of (a) molarity, (b) molality.
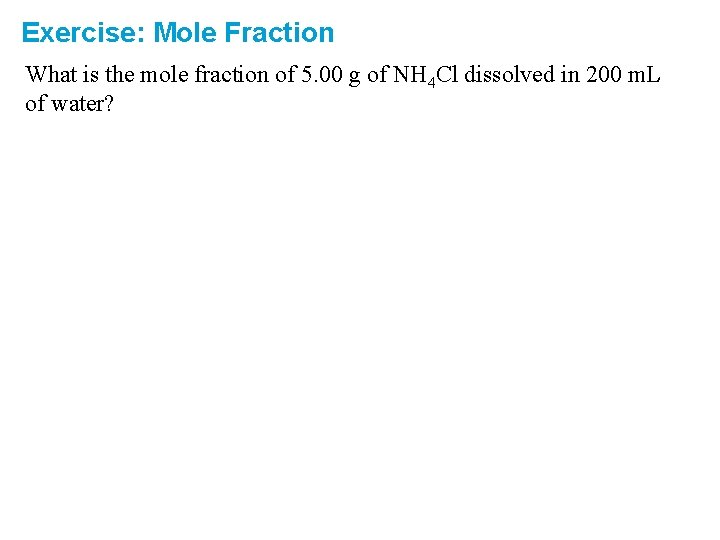
Exercise: Mole Fraction What is the mole fraction of 5. 00 g of NH 4 Cl dissolved in 200 m. L of water?
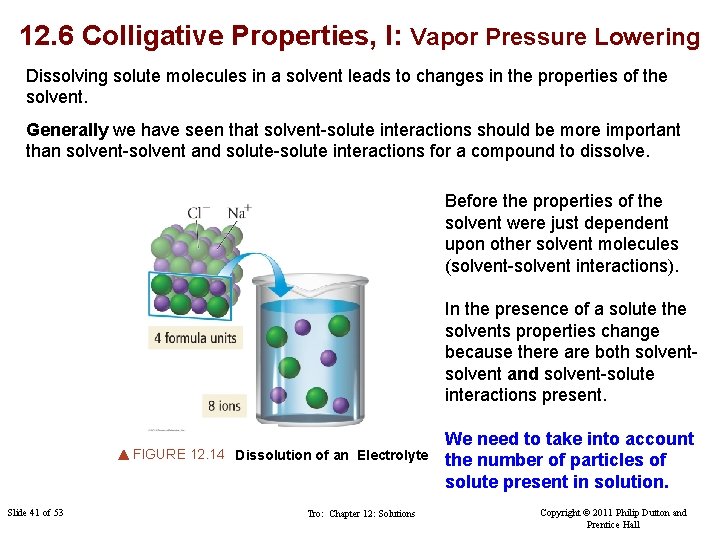
12. 6 Colligative Properties, I: Vapor Pressure Lowering Dissolving solute molecules in a solvent leads to changes in the properties of the solvent. Generally we have seen that solvent-solute interactions should be more important than solvent-solvent and solute-solute interactions for a compound to dissolve. Before the properties of the solvent were just dependent upon other solvent molecules (solvent-solvent interactions). In the presence of a solute the solvents properties change because there are both solvent and solvent-solute interactions present. FIGURE 12. 14 Dissolution of an Electrolyte Slide 41 of 53 Tro: Chapter 12: Solutions We need to take into account the number of particles of solute present in solution. Copyright © 2011 Philip Dutton and Prentice Hall
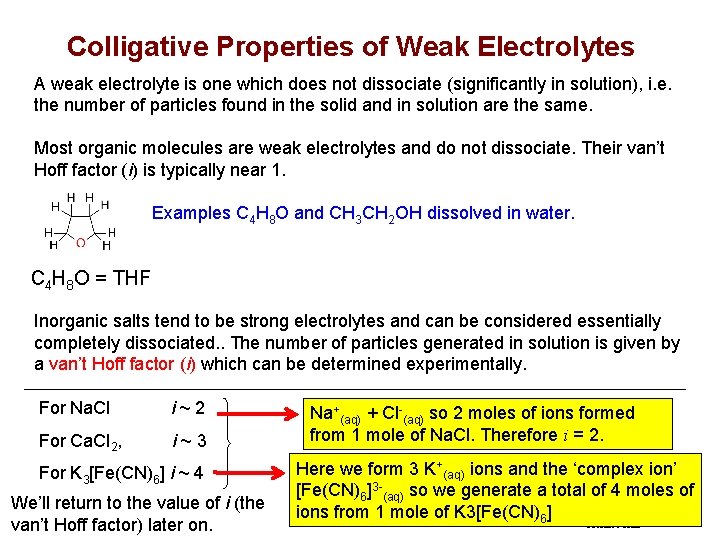
Colligative Properties of Weak Electrolytes A weak electrolyte is one which does not dissociate (significantly in solution), i. e. the number of particles found in the solid and in solution are the same. Most organic molecules are weak electrolytes and do not dissociate. Their van’t Hoff factor (i) is typically near 1. Examples C 4 H 8 O and CH 3 CH 2 OH dissolved in water. C 4 H 8 O = THF Inorganic salts tend to be strong electrolytes and can be considered essentially completely dissociated. . The number of particles generated in solution is given by a van’t Hoff factor (i) which can be determined experimentally. For Na. Cl For Ca. Cl 2, i~2 i~3 FIGURE For K 3[Fe(CN)6] i ~ 4 We’ll return to the value of i (the van’t Hoff factor) later on. Slide 42 of 53 Na+(aq) + Cl-(aq) so 2 moles of ions formed from 1 mole of Na. Cl. Therefore i = 2. Here we form 3 K+(aq) ions and the ‘complex ion’ [Fe(CN)6]3 -(aq) so we generate a total of 4 moles of © 2011 Philip Dutton and Tro: Chapter ions from 12: 1 Solutions mole of K 3[Fe(CN)Copyright 6] Prentice Hall
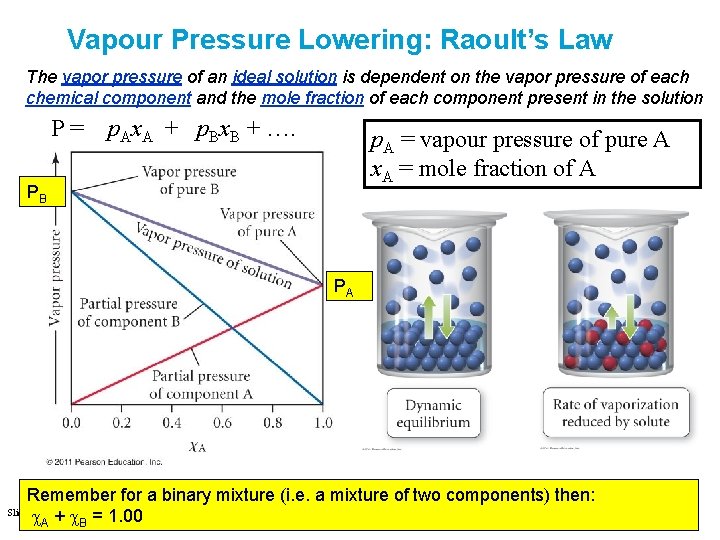
Vapour Pressure Lowering: Raoult’s Law The vapor pressure of an ideal solution is dependent on the vapor pressure of each chemical component and the mole fraction of each component present in the solution P= p. Ax. A + p. Bx. B + …. p. A = vapour pressure of pure A x. A = mole fraction of A PB PA Remember for a binary mixture (i. e. a mixture of two components) then: Slide 43 of 53 Tro: Chapter 12: Solutions c. A + c. B = 1. 00
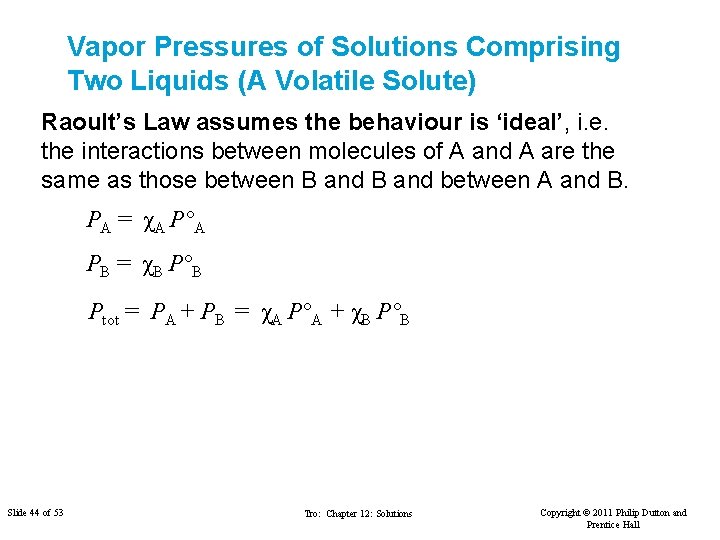
Vapor Pressures of Solutions Comprising Two Liquids (A Volatile Solute) Raoult’s Law assumes the behaviour is ‘ideal’, i. e. the interactions between molecules of A and A are the same as those between B and between A and B. PA = χA P°A PB = χB P°B Ptot = PA + PB = χA P°A + χB P°B Slide 44 of 53 Tro: Chapter 12: Solutions Copyright © 2011 Philip Dutton and Prentice Hall
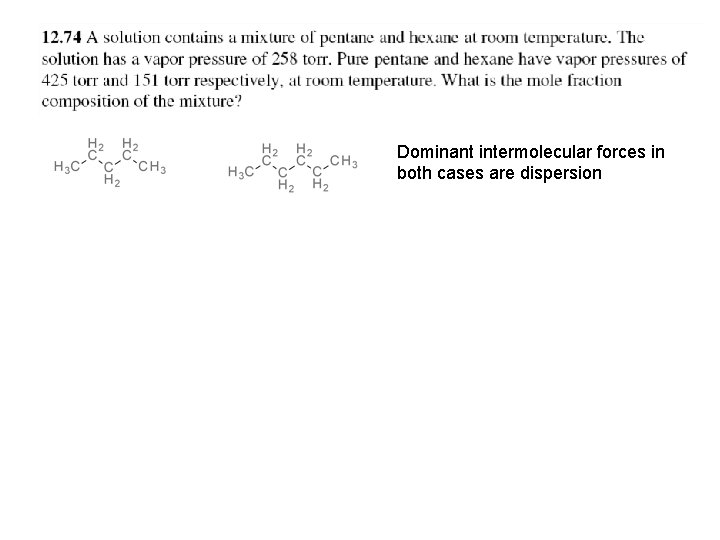
Dominant intermolecular forces in both cases are dispersion
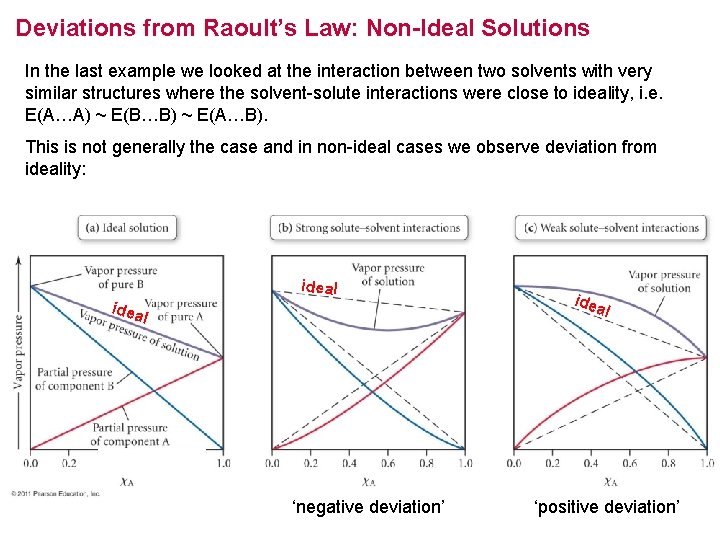
Deviations from Raoult’s Law: Non-Ideal Solutions In the last example we looked at the interaction between two solvents with very similar structures where the solvent-solute interactions were close to ideality, i. e. E(A…A) ~ E(B…B) ~ E(A…B). This is not generally the case and in non-ideal cases we observe deviation from ideality: ideal idea l FIGURE ‘negative deviation’ ‘positive deviation’
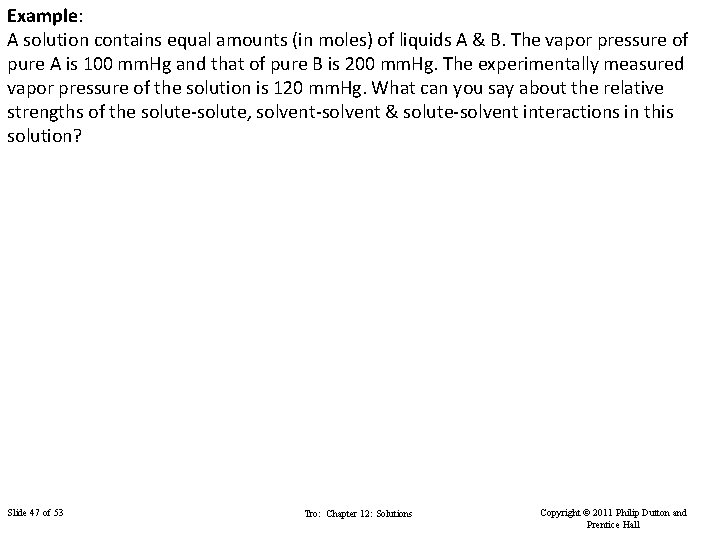
Example: A solution contains equal amounts (in moles) of liquids A & B. The vapor pressure of pure A is 100 mm. Hg and that of pure B is 200 mm. Hg. The experimentally measured vapor pressure of the solution is 120 mm. Hg. What can you say about the relative strengths of the solute-solute, solvent-solvent & solute-solvent interactions in this solution? FIGURE Slide 47 of 53 Tro: Chapter 12: Solutions Copyright © 2011 Philip Dutton and Prentice Hall
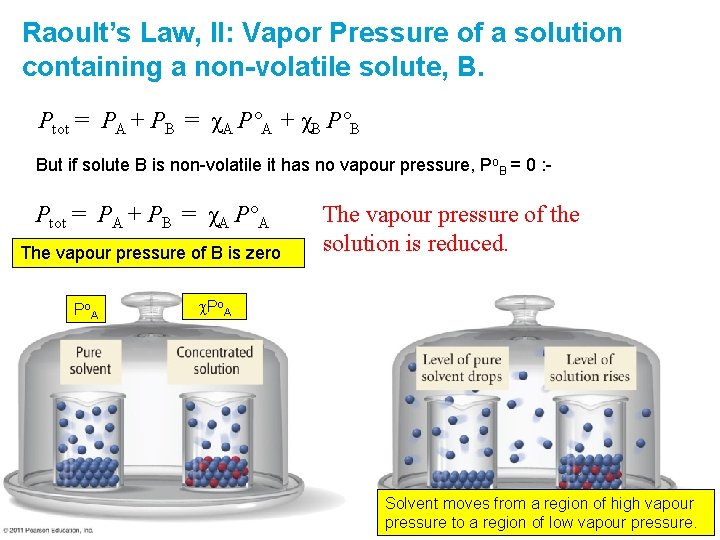
Raoult’s Law, II: Vapor Pressure of a solution containing a non-volatile solute, B. Ptot = PA + PB = χA P°A + χB P°B But if solute B is non-volatile it has no vapour pressure, Po. B = 0 : - Ptot = PA + PB = χA P°A The vapour pressure of B is zero P o. A The vapour pressure of the solution is reduced. c. Po. A Solvent moves from a region of high vapour pressure to a region of low vapour pressure.
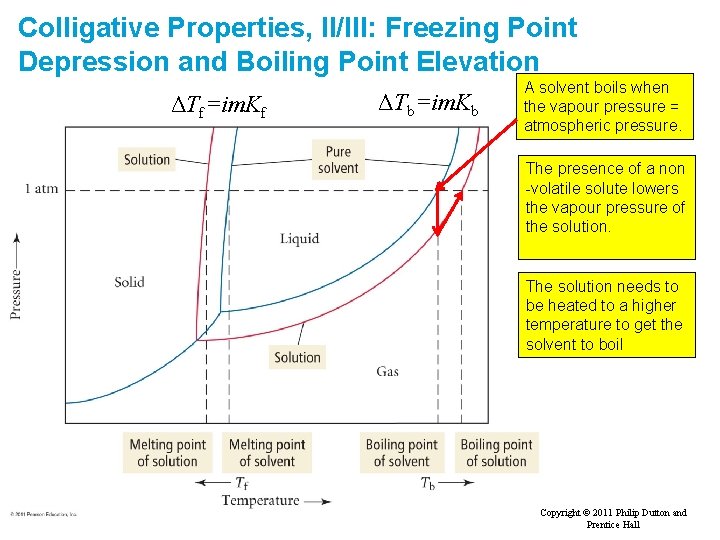
Colligative Properties, II/III: Freezing Point Depression and Boiling Point Elevation ΔTf=im. Kf ΔTb=im. Kb A solvent boils when the vapour pressure = atmospheric pressure. The presence of a non -volatile solute lowers the vapour pressure of the solution. The solution needs to be heated to a higher temperature to get the solvent to boil Slide 49 of 53 Tro: Chapter 12: Solutions Copyright © 2011 Philip Dutton and Prentice Hall
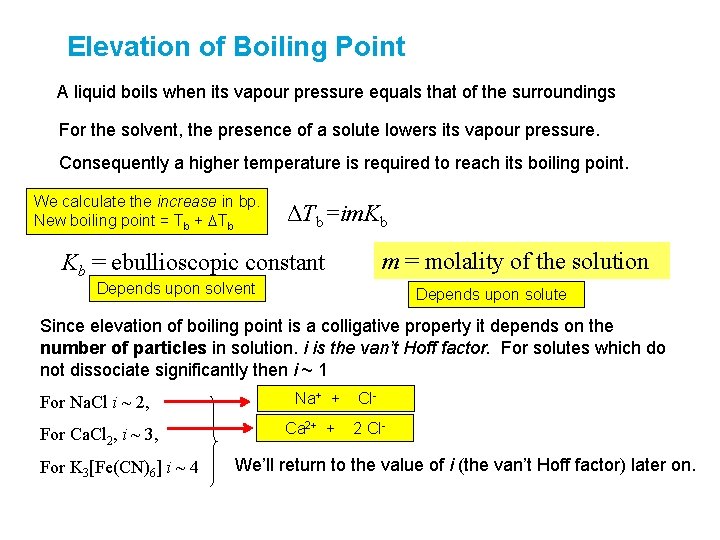
Elevation of Boiling Point A liquid boils when its vapour pressure equals that of the surroundings For the solvent, the presence of a solute lowers its vapour pressure. Consequently a higher temperature is required to reach its boiling point. We calculate the increase in bp. New boiling point = Tb + DTb ΔTb=im. Kb m = molality of the solution Kb = ebullioscopic constant Depends upon solvent Depends upon solute Since elevation of boiling point is a colligative property it depends on the number of particles in solution. i is the van’t Hoff factor. For solutes which do not dissociate significantly then i ~ 1 For Na. Cl i ~ 2, For Ca. Cl 2, i ~ 3, For K 3[Fe(CN)6] i ~ 4 Na+ + Ca 2+ + Cl 2 Cl- We’ll return to the value of i (the van’t Hoff factor) later on.
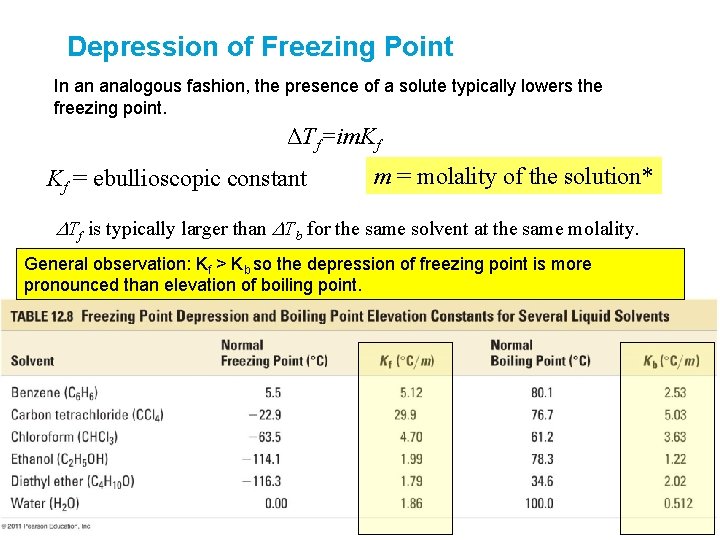
Depression of Freezing Point In an analogous fashion, the presence of a solute typically lowers the freezing point. ΔTf=im. Kf Kf = ebullioscopic constant m = molality of the solution* DTf is typically larger than DTb for the same solvent at the same molality. General observation: Kf > Kb so the depression of freezing point is more pronounced than elevation of boiling point.
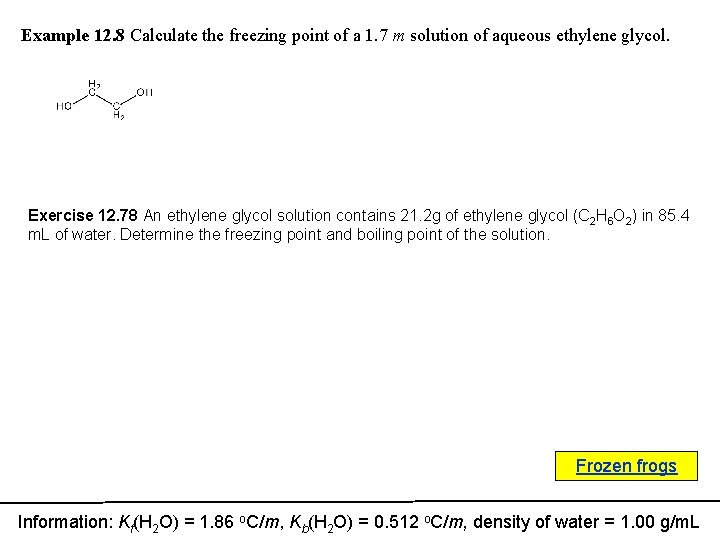
Example 12. 8 Calculate the freezing point of a 1. 7 m solution of aqueous ethylene glycol. Exercise 12. 78 An ethylene glycol solution contains 21. 2 g of ethylene glycol (C 2 H 6 O 2) in 85. 4 m. L of water. Determine the freezing point and boiling point of the solution. Frozen frogs Information: Kf(H 2 O) = 1. 86 o. C/m, Kb(H 2 O) = 0. 512 o. C/m, density of water = 1. 00 g/m. L
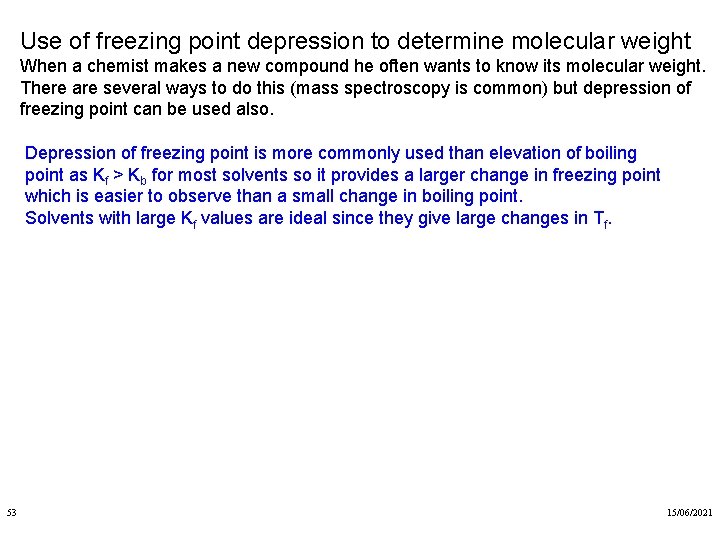
Use of freezing point depression to determine molecular weight When a chemist makes a new compound he often wants to know its molecular weight. There are several ways to do this (mass spectroscopy is common) but depression of freezing point can be used also. Depression of freezing point is more commonly used than elevation of boiling point as Kf > Kb for most solvents so it provides a larger change in freezing point which is easier to observe than a small change in boiling point. Solvents with large Kf values are ideal since they give large changes in Tf. 53 15/06/2021
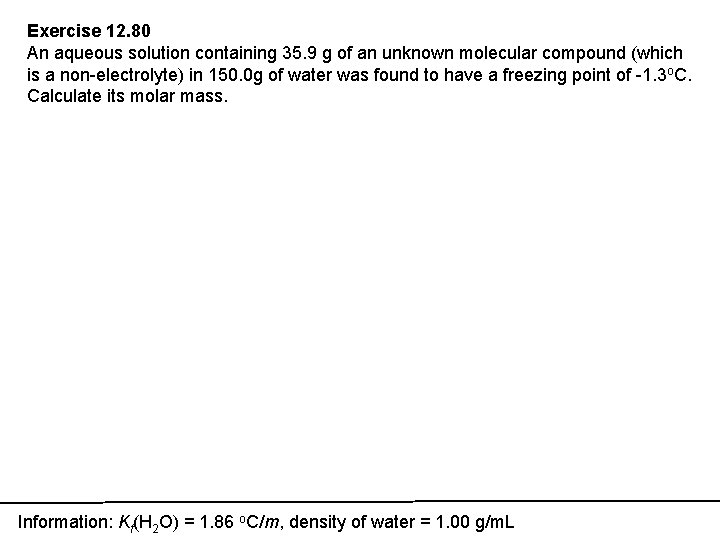
Exercise 12. 80 An aqueous solution containing 35. 9 g of an unknown molecular compound (which is a non-electrolyte) in 150. 0 g of water was found to have a freezing point of -1. 3 o. C. Calculate its molar mass. Information: Kf(H 2 O) = 1. 86 o. C/m, density of water = 1. 00 g/m. L
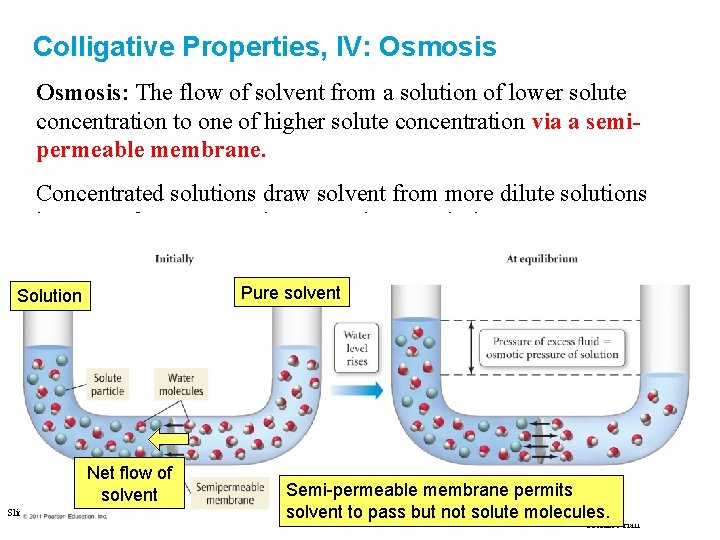
Colligative Properties, IV: Osmosis: The flow of solvent from a solution of lower solute concentration to one of higher solute concentration via a semipermeable membrane. Concentrated solutions draw solvent from more dilute solutions because of nature’s tendency to mix – maximises entropy. Pure solvent Solution Net flow of solvent Slide 55 of 53 Semi-permeable membrane permits Copyright © 2011 Philip Dutton and Tro: Chapter Solutionsbut not solute molecules. solvent to 12: pass Prentice Hall
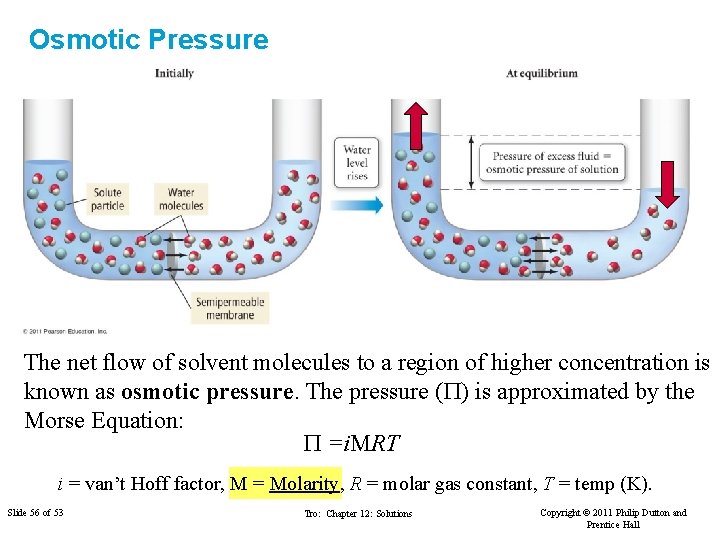
Osmotic Pressure The net flow of solvent molecules to a region of higher concentration is known as osmotic pressure. The pressure (P) is approximated by the Morse Equation: P =i. MRT i = van’t Hoff factor, M = Molarity, R = molar gas constant, T = temp (K). Slide 56 of 53 Tro: Chapter 12: Solutions Copyright © 2011 Philip Dutton and Prentice Hall
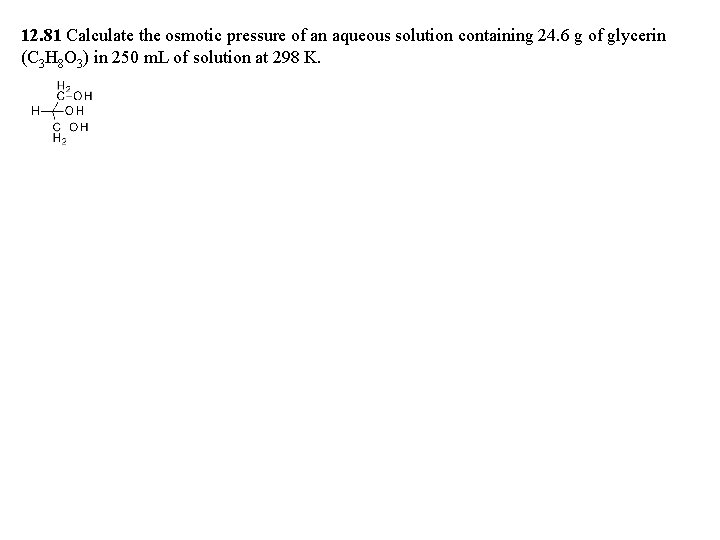
12. 81 Calculate the osmotic pressure of an aqueous solution containing 24. 6 g of glycerin (C 3 H 8 O 3) in 250 m. L of solution at 298 K.
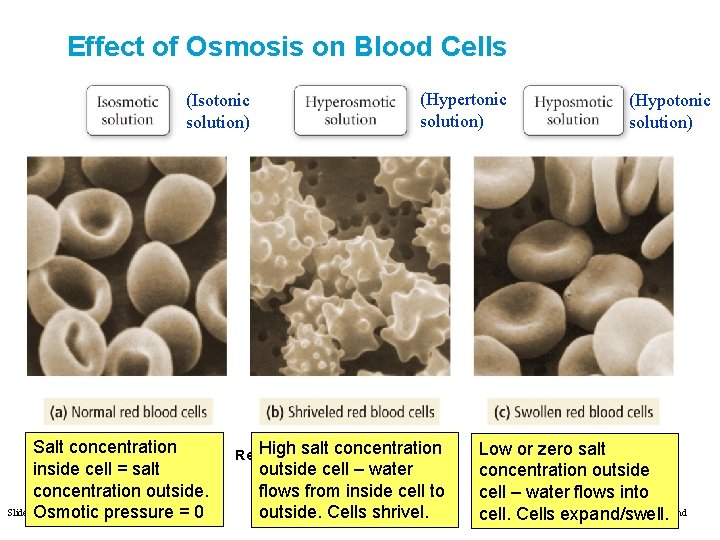
Effect of Osmosis on Blood Cells (Isotonic solution) Salt concentration FIGURE 12. 18 inside cell = salt concentration outside. Slide 58 of 53 Osmotic pressure = 0 (Hypertonic solution) concentration Red. High Bloodsalt Cells and Osmosis outside cell – water flows from inside cell to Tro: Cells Chapter 12: shrivel. Solutions outside. (Hypotonic solution) Low or zero salt concentration outside cell – water flows into Copyright © 2011 Philip Dutton and cell. Cells expand/swell. Prentice Hall
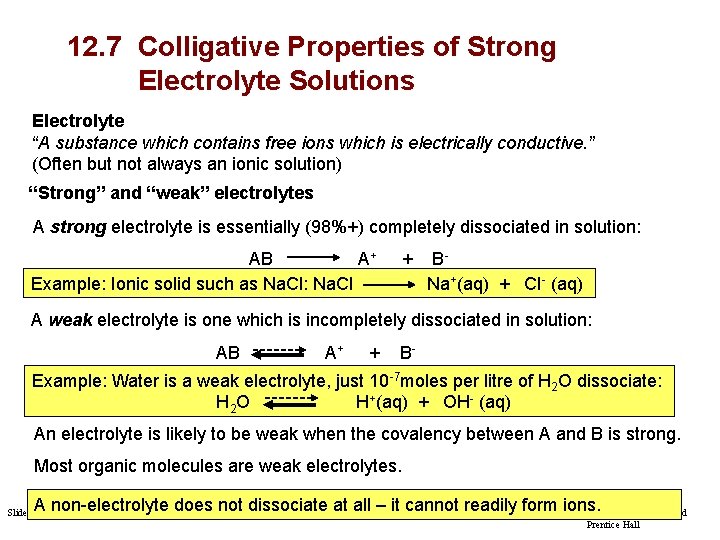
12. 7 Colligative Properties of Strong Electrolyte Solutions Electrolyte “A substance which contains free ions which is electrically conductive. ” (Often but not always an ionic solution) “Strong” and “weak” electrolytes A strong electrolyte is essentially (98%+) completely dissociated in solution: AB A+ Example: Ionic solid such as Na. Cl: Na. Cl + BNa+(aq) + Cl- (aq) A weak electrolyte is one which is incompletely dissociated in solution: AB A+ + B- Example: Water is a weak electrolyte, just 10 -7 moles per litre of H 2 O dissociate: H 2 O H+(aq) + OH- (aq) An electrolyte is likely to be weak when the covalency between A and B is strong. Most organic molecules are weak electrolytes. A non-electrolyte does not dissociate at all – it cannot readily form ions. Copyright © 2011 Philip Dutton and Tro: Chapter 12: Solutions Slide 59 of 53 Prentice Hall
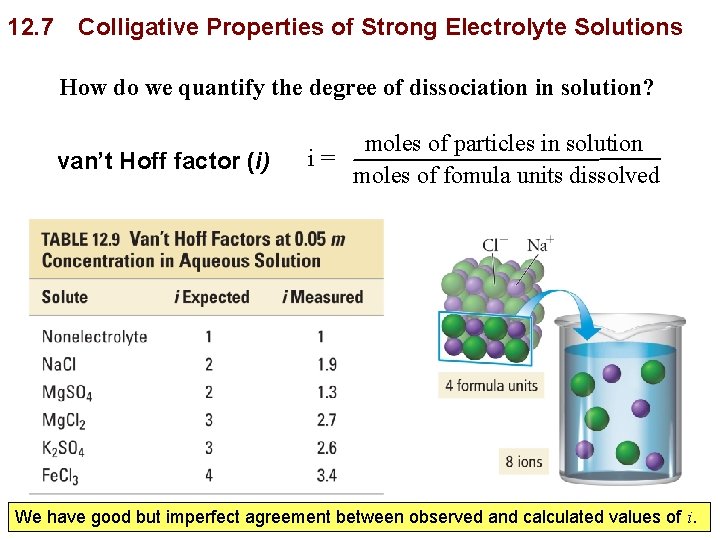
12. 7 Colligative Properties of Strong Electrolyte Solutions How do we quantify the degree of dissociation in solution? van’t Hoff factor (i) moles of particles in solution i= moles of fomula units dissolved Copyright © 2011 Philip Dutton and Tro: Chapter 12: Solutions We have good but imperfect agreement between observed and calculated values of i. Prentice Hall Slide 60 of 53
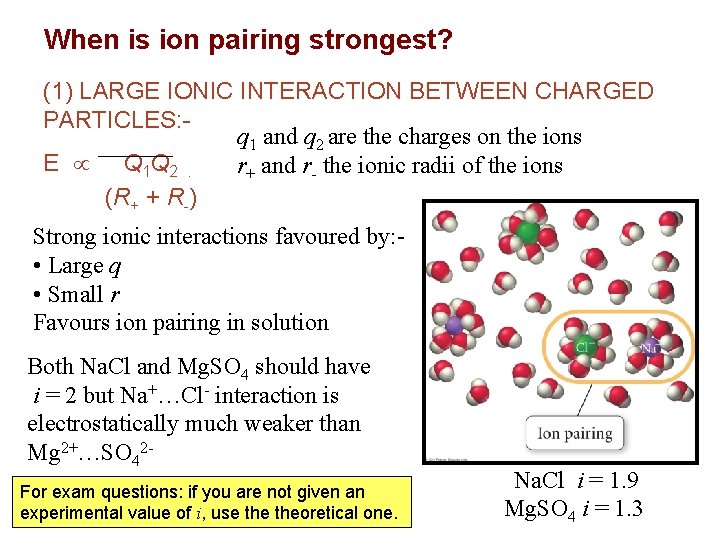
When is ion pairing strongest? (1) LARGE IONIC INTERACTION BETWEEN CHARGED PARTICLES: q 1 and q 2 are the charges on the ions E Q 1 Q 2. r+ and r- the ionic radii of the ions (R+ + R-) Strong ionic interactions favoured by: • Large q • Small r Favours ion pairing in solution Both Na. Cl and Mg. SO 4 should have i = 2 but Na+…Cl- interaction is electrostatically much weaker than 2 Mg 2+…SO 4 FIGURE For exam questions: if you are not given an experimental value of i, use theoretical one. Na. Cl i = 1. 9 Mg. SO 4 i = 1. 3
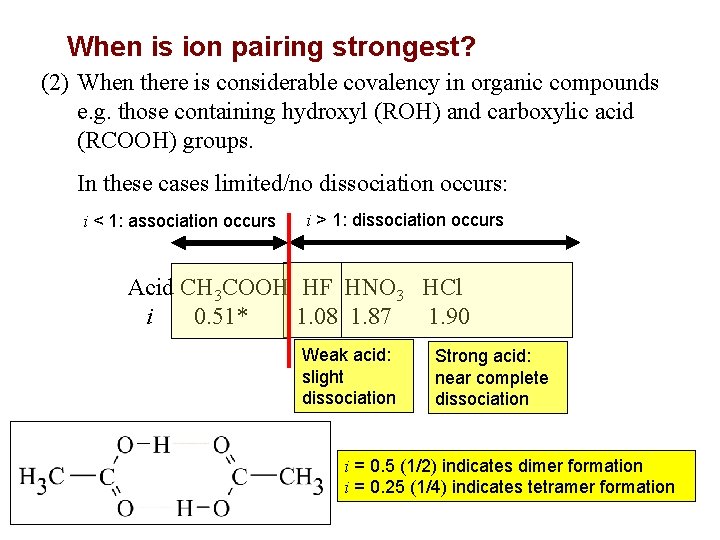
When is ion pairing strongest? (2) When there is considerable covalency in organic compounds e. g. those containing hydroxyl (ROH) and carboxylic acid (RCOOH) groups. In these cases limited/no dissociation occurs: i < 1: association occurs i > 1: dissociation occurs Acid CH 3 COOH HF HNO 3 HCl i 0. 51* 1. 08 1. 87 1. 90 Weak acid: slight dissociation FIGURE Strong acid: near complete dissociation i = 0. 5 (1/2) indicates dimer formation i = 0. 25 (1/4) indicates tetramer formation
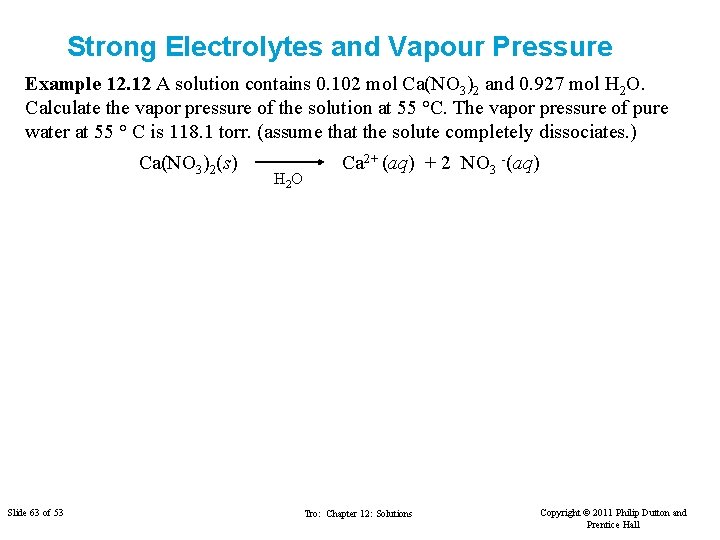
Strong Electrolytes and Vapour Pressure Example 12. 12 A solution contains 0. 102 mol Ca(NO 3)2 and 0. 927 mol H 2 O. Calculate the vapor pressure of the solution at 55 °C. The vapor pressure of pure water at 55 ° C is 118. 1 torr. (assume that the solute completely dissociates. ) Ca(NO 3)2(s) Slide 63 of 53 H 2 O Ca 2+ (aq) + 2 NO 3 -(aq) Tro: Chapter 12: Solutions Copyright © 2011 Philip Dutton and Prentice Hall
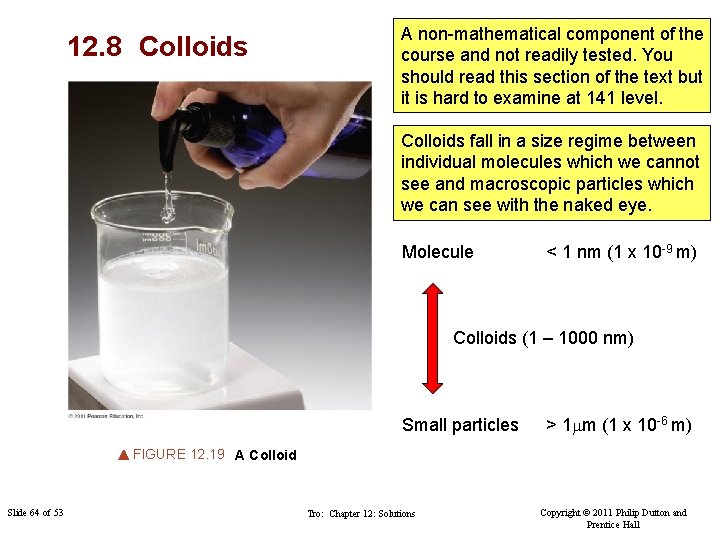
12. 8 Colloids A non-mathematical component of the course and not readily tested. You should read this section of the text but it is hard to examine at 141 level. Colloids fall in a size regime between individual molecules which we cannot see and macroscopic particles which we can see with the naked eye. Molecule < 1 nm (1 x 10 -9 m) Colloids (1 – 1000 nm) Small particles > 1 mm (1 x 10 -6 m) FIGURE 12. 19 A Colloid Slide 64 of 53 Tro: Chapter 12: Solutions Copyright © 2011 Philip Dutton and Prentice Hall
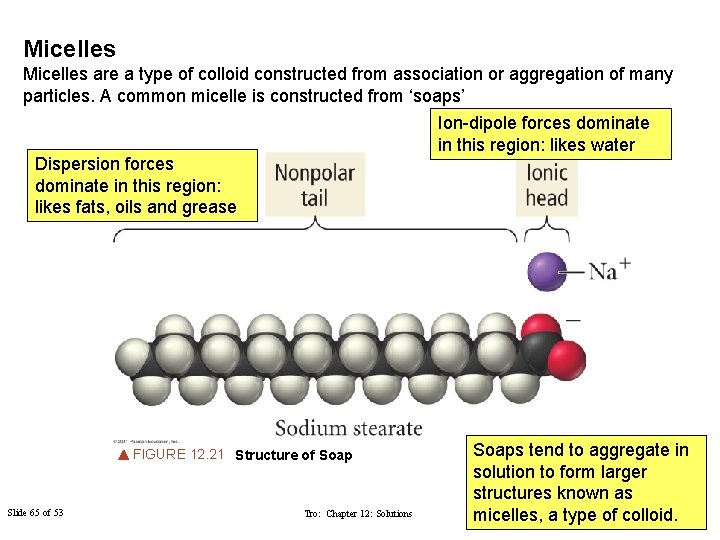
Micelles are a type of colloid constructed from association or aggregation of many particles. A common micelle is constructed from ‘soaps’ Ion-dipole forces dominate in this region: likes water Dispersion forces dominate in this region: likes fats, oils and grease FIGURE 12. 21 Structure of Soap Slide 65 of 53 Tro: Chapter 12: Solutions Soaps tend to aggregate in solution to form larger structures known as © 2011 Dutton and micelles, Copyright a type of. Philip colloid. Prentice Hall
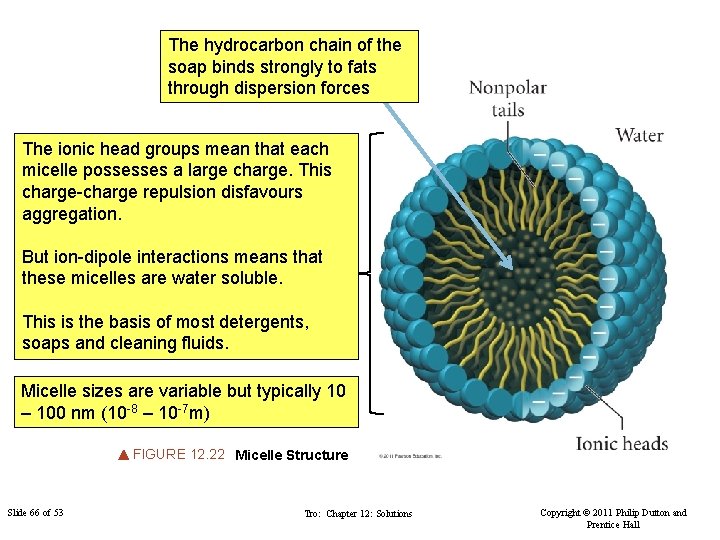
The hydrocarbon chain of the soap binds strongly to fats through dispersion forces The ionic head groups mean that each micelle possesses a large charge. This charge-charge repulsion disfavours aggregation. But ion-dipole interactions means that these micelles are water soluble. This is the basis of most detergents, soaps and cleaning fluids. Micelle sizes are variable but typically 10 – 100 nm (10 -8 – 10 -7 m) FIGURE 12. 22 Micelle Structure Slide 66 of 53 Tro: Chapter 12: Solutions Copyright © 2011 Philip Dutton and Prentice Hall
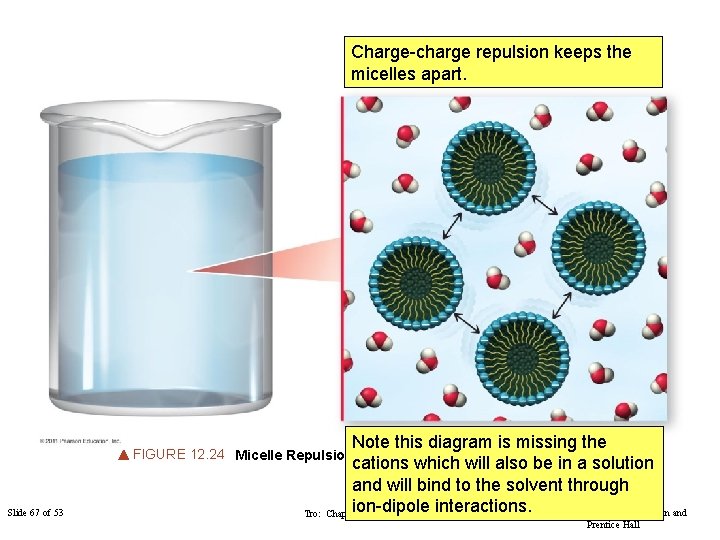
Charge-charge repulsion keeps the micelles apart. Note this diagram is missing the cations which will also be in a solution and will bind to the solvent through ion-dipole interactions. Copyright © 2011 Philip Dutton and Tro: Chapter 12: Solutions FIGURE 12. 24 Micelle Repulsion Slide 67 of 53 Prentice Hall
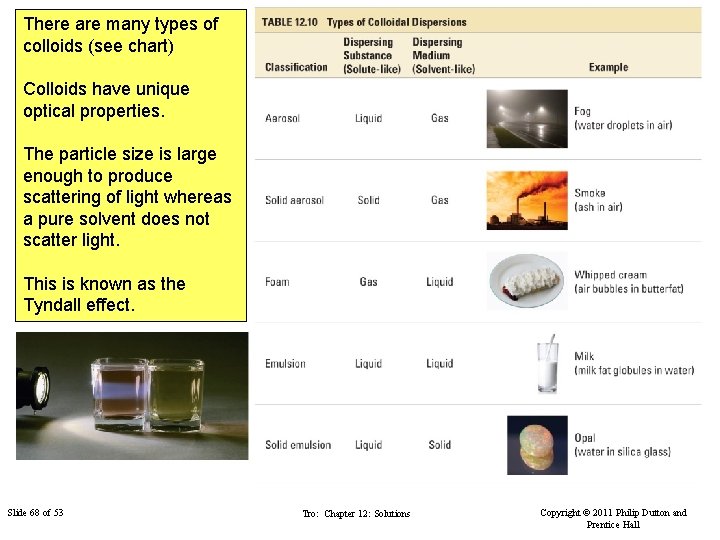
There are many types of colloids (see chart) Colloids have unique optical properties. The particle size is large enough to produce scattering of light whereas a pure solvent does not scatter light. This is known as the Tyndall effect. Slide 68 of 53 Tro: Chapter 12: Solutions Copyright © 2011 Philip Dutton and Prentice Hall
Prefix multipliers
Introductory chemistry 5th edition nivaldo j. tro
Nivaldo j. tro introductory chemistry
Jack baldermann westmont high school
Democritus atomic model diagram
Covalent bond melting point
Ionic covalent metallic
Giant molecular structure vs simple molecular structure
Ap chemistry molecular geometry
Notice tro
Bra bre bri bro bru
Bài 2 cải tạo tu bổ vườn tạp
Hỡi người hãy nhớ mình là bụi tro
Tò vò mà nuôi con nhện phương thức biểu đạt
Con trỏ sql
Trò chơi khuông nhạc bàn tay
Trò chơi xem kịch câm
Trò chơi chữ cái u ư chủ đề nghề nghiệp
1 tro
Tro
Humility in bible means
Bài tập về nhà
Bài hát ước mơ lớp 5
Tro
Vai trò của thực vật đối với động vật
Vai trò thực tiễn của lớp giáp xác
Aftalers ugyldighed
ơn phù trợ chúng ta ở nơi danh chúa
Trả lời câu hỏi nghĩa thầy trò
Ib organic chemistry
Inorganic chemistry vs organic chemistry
Approach chemistry chalk chapter
Virtual circuits and datagram networks
Theoretical models of counseling
Shower approach marketing
Multiple approach-avoidance
Cognitive approach vs behavioral approach
What is a research
Diagram for traditional approach
Deep learning approach and surface learning approach
Vsepr
Chemistry geometric shapes
Ax3e molecular shape
Molecular biology crash course
Molecular level vs cellular level
Molecular absorption
Sf5cl lewis structure
Kenitic molecular theory
Covalent molecular and covalent network
Kinetic molecular theory of liquids and solids
The chemical formula of a compound indicates
Criterios de amsterdam cancer de colon
Molecular geometry chart
Palindromic sequence
Molecular rebar
Covalent molecular substances
Empirical formula of haemoglobin
Polydispersity
Molecular clock theory
How to find molecular formula
Molecular mass of potassium permanganate
Empirical formula to percent composition
How to calculate percent composition by mass
Molecular modelling laboratory
Molecular replacement method
Cycloalkanes
Naming compounds and writing formulas
Binary molecular
N srinivasan mbu iisc