Chapter 19 Eukaryotic Genomes Organization Regulation and Evolution
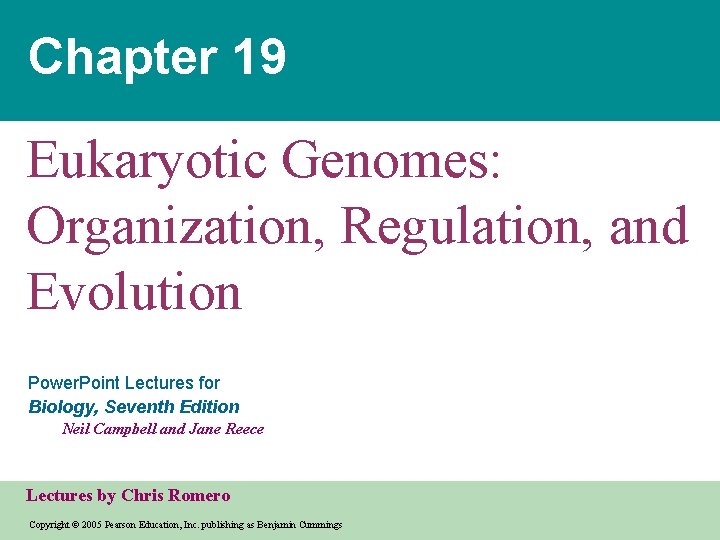
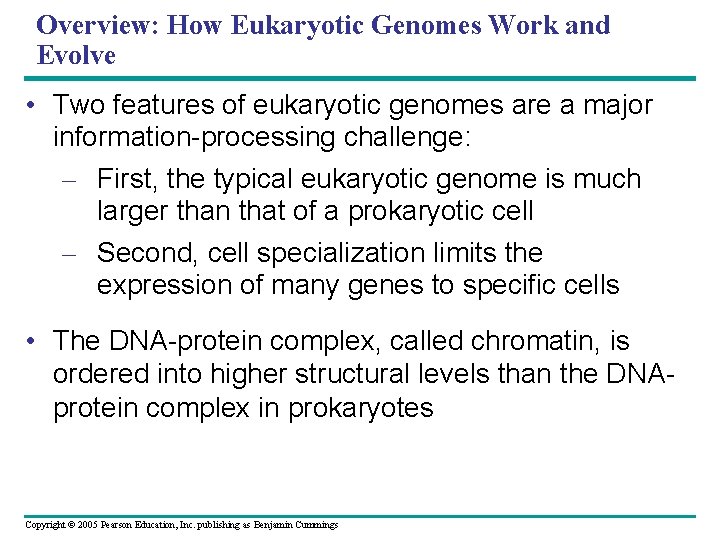
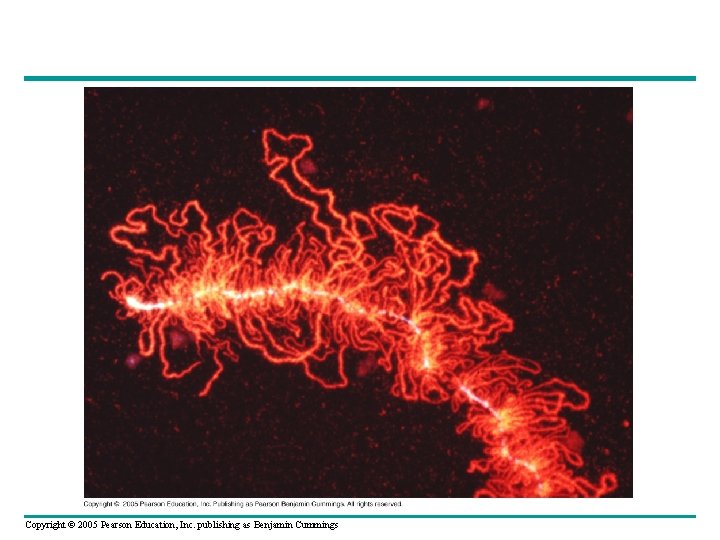
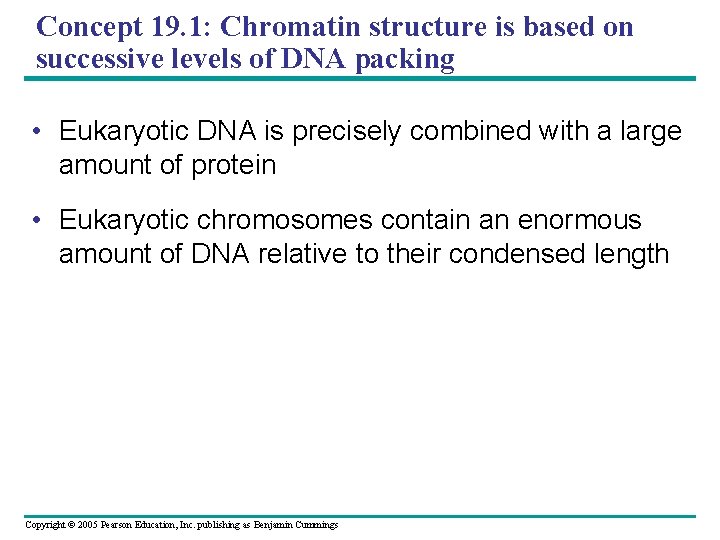
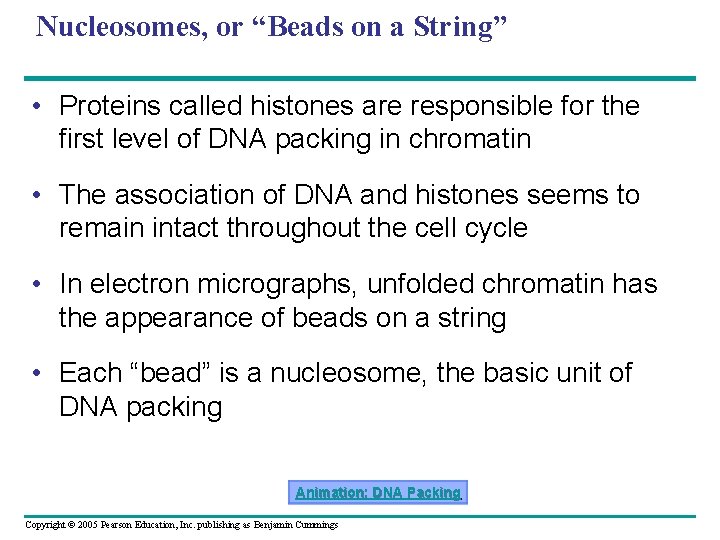
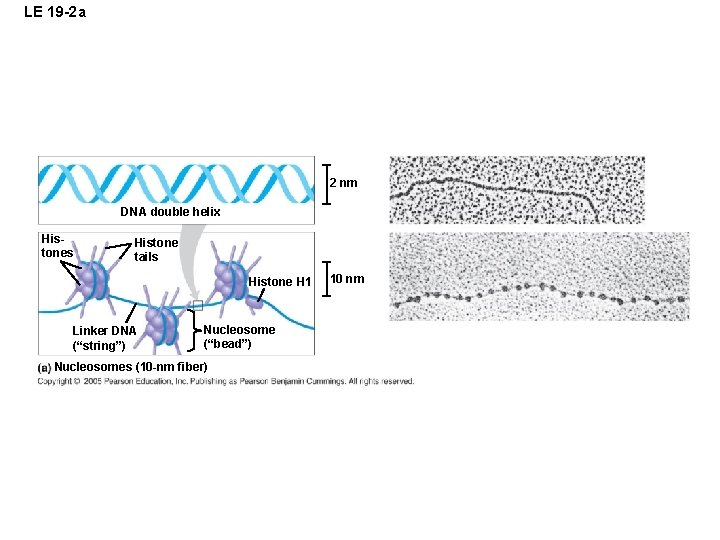
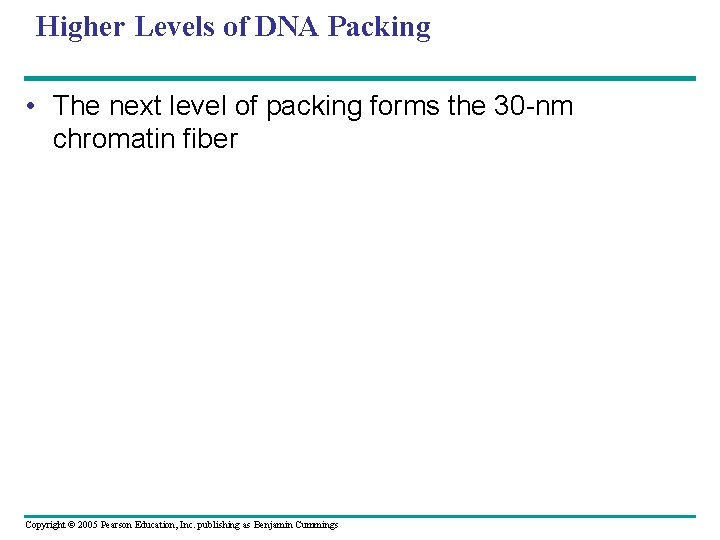
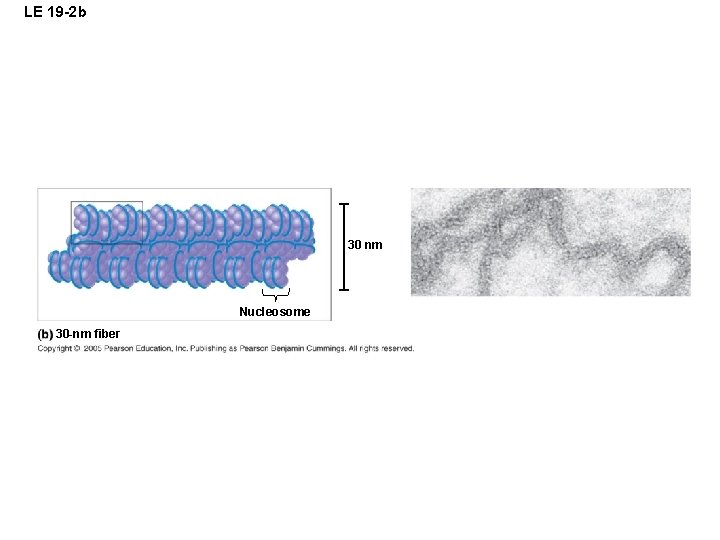
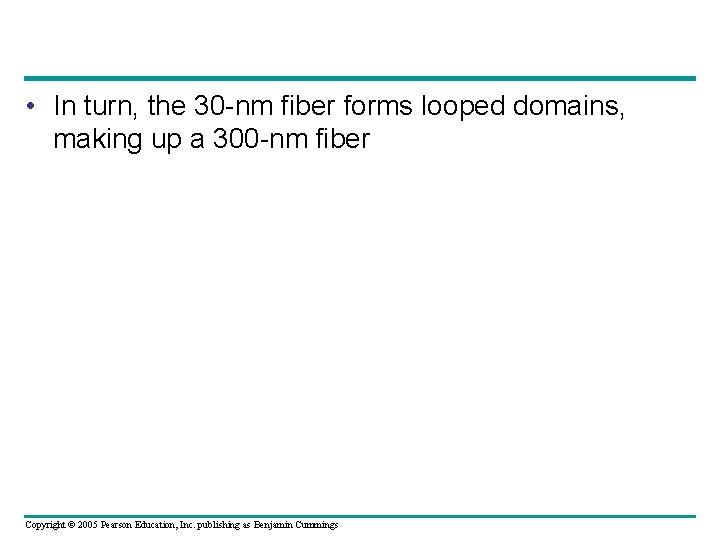
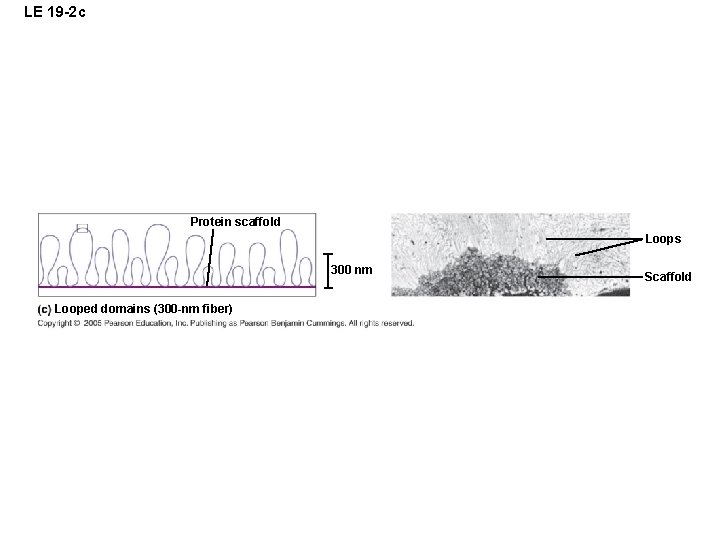
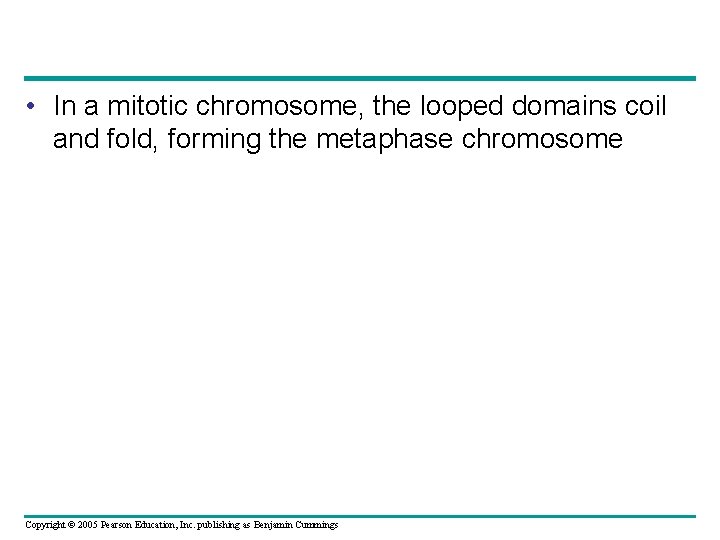
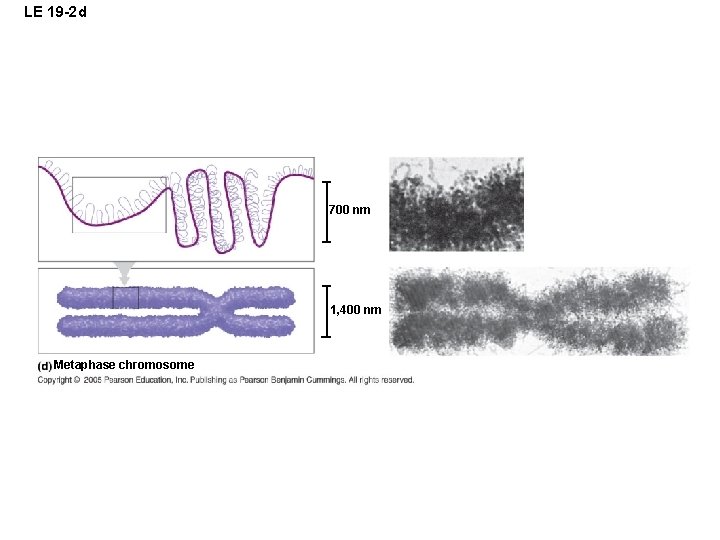
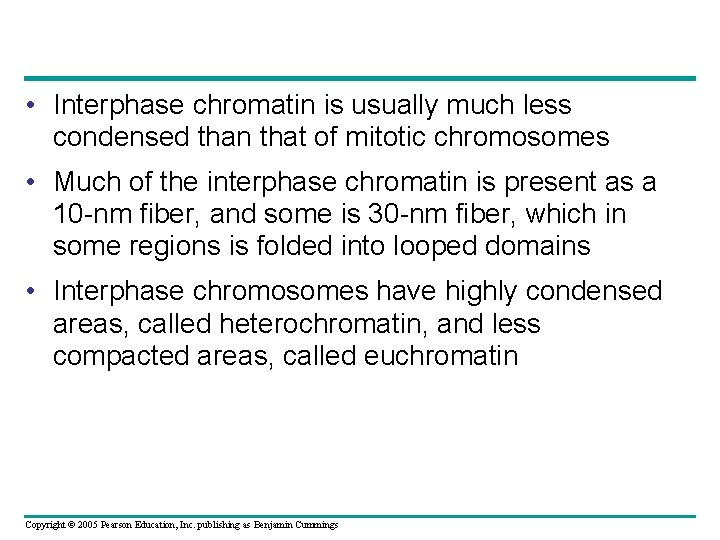
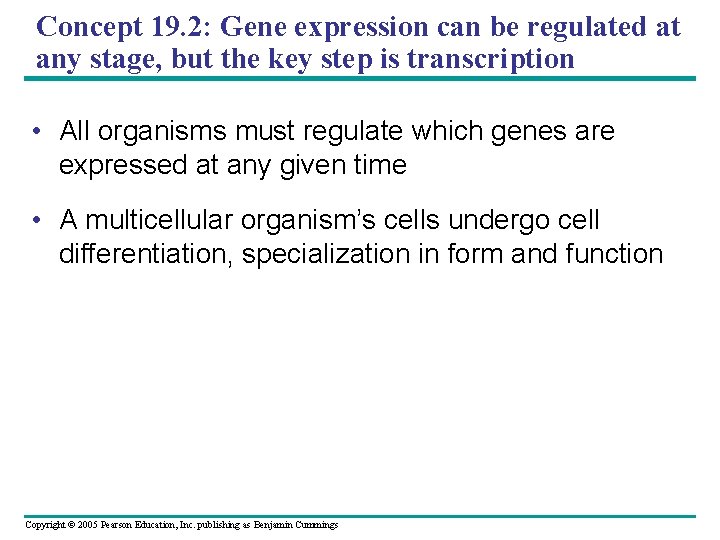
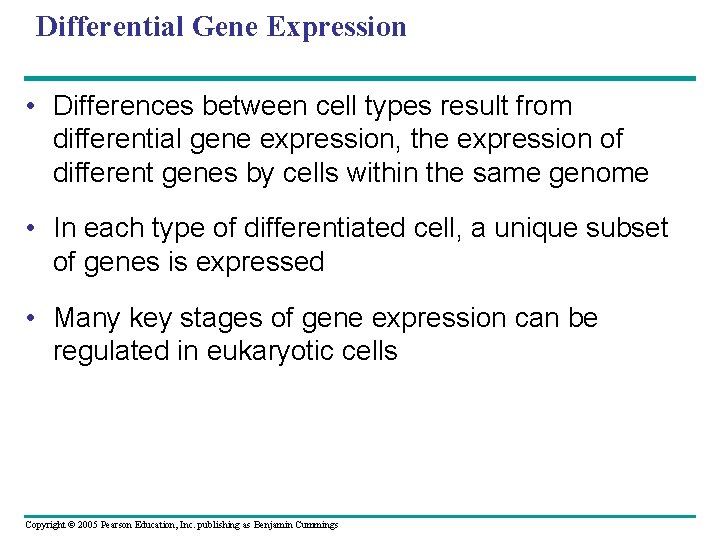
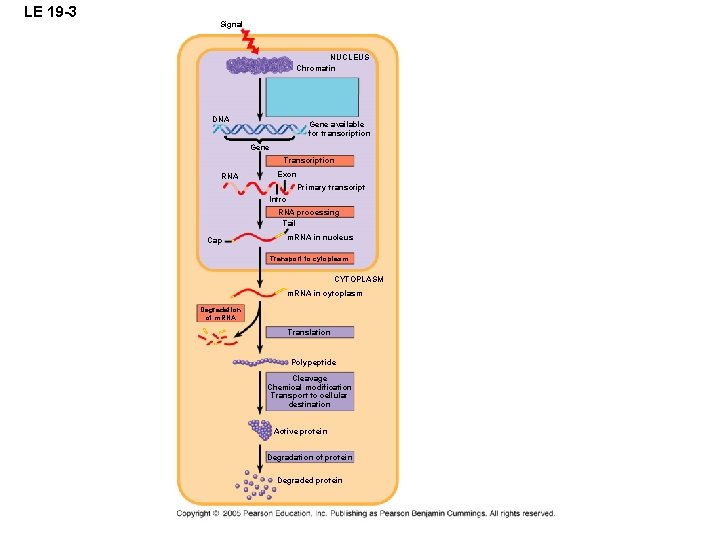
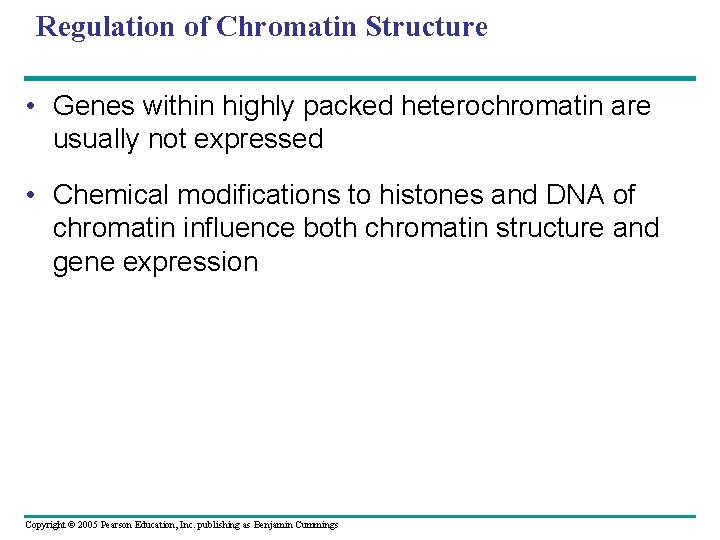
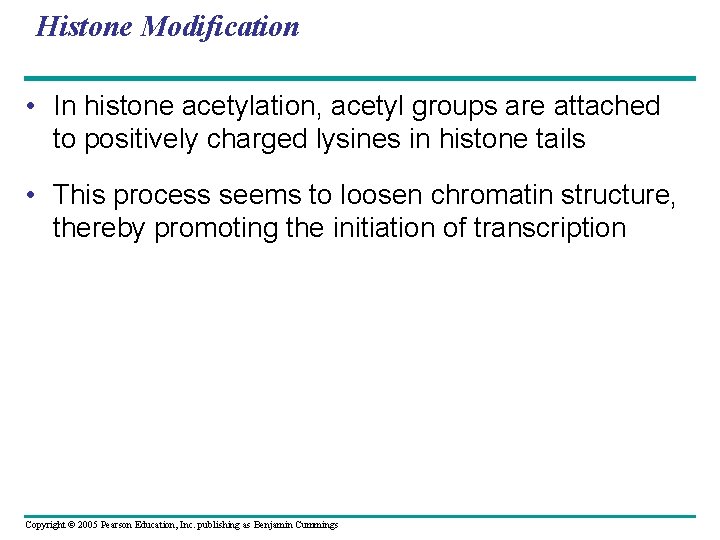
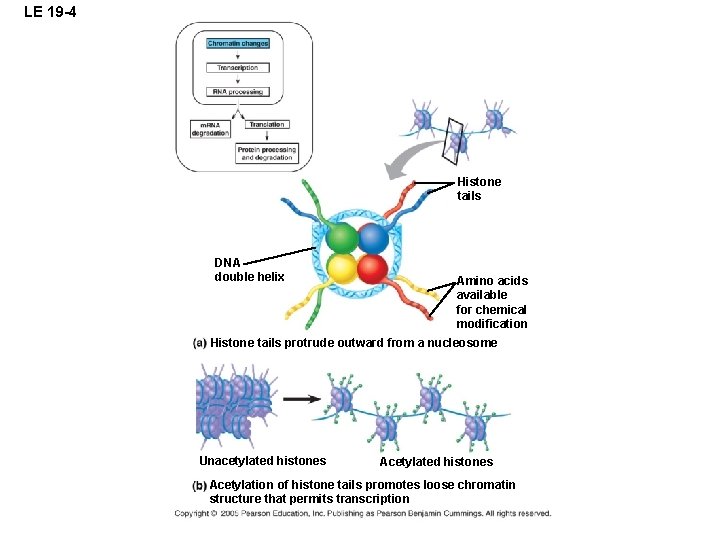
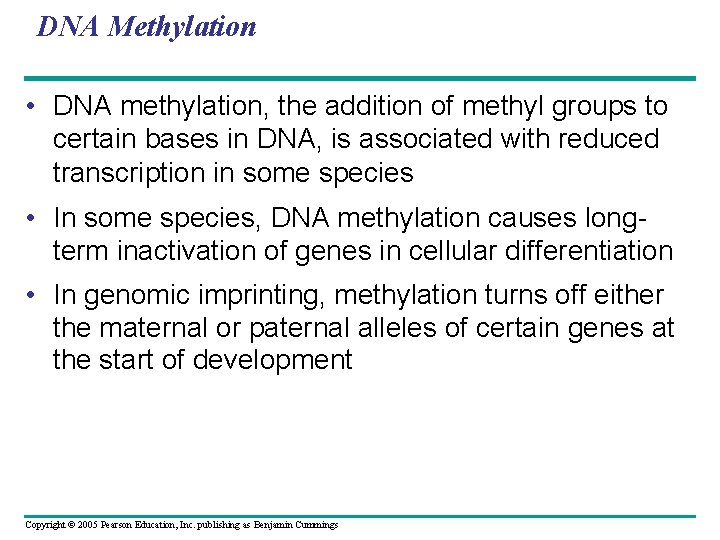
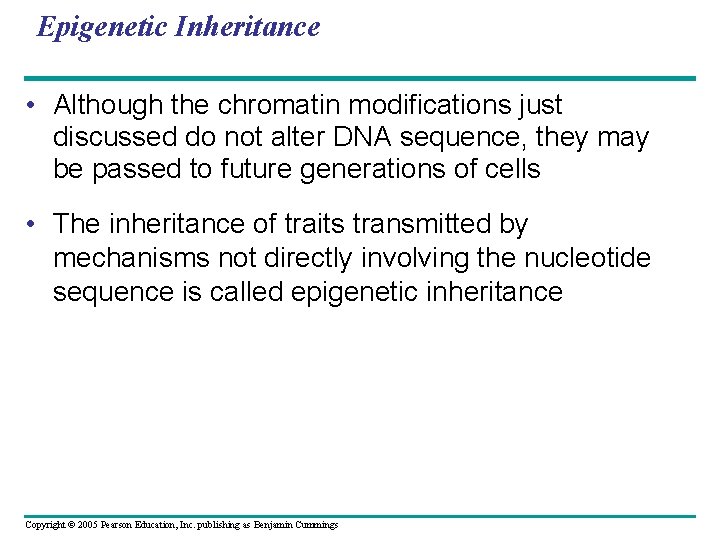
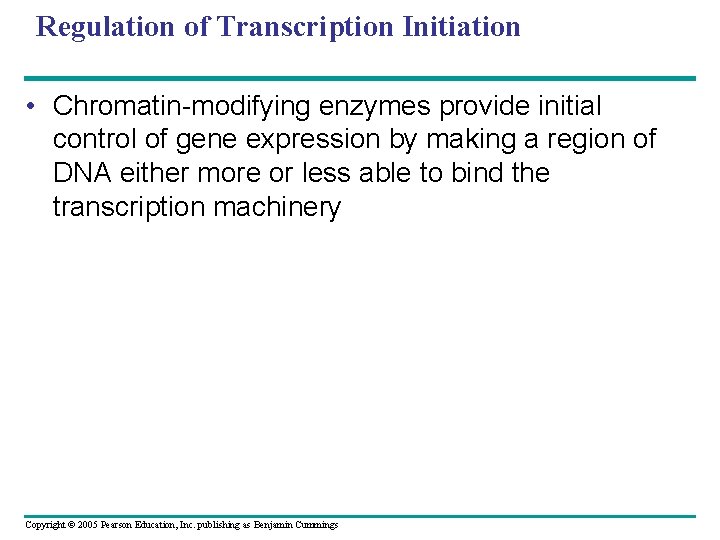
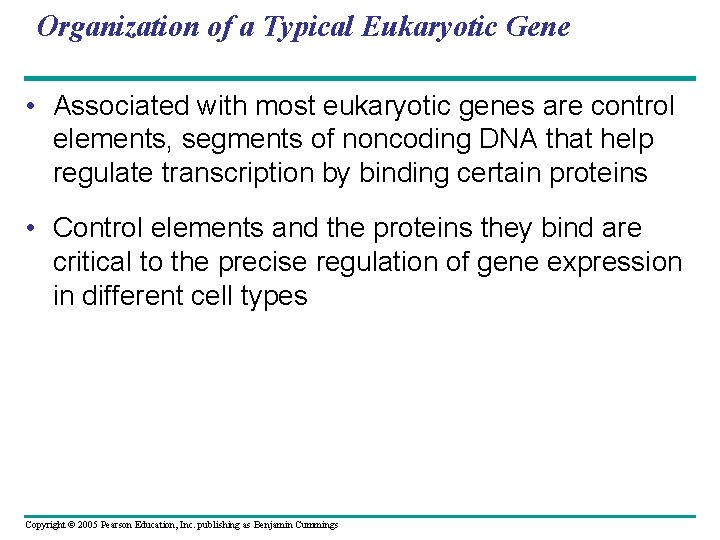
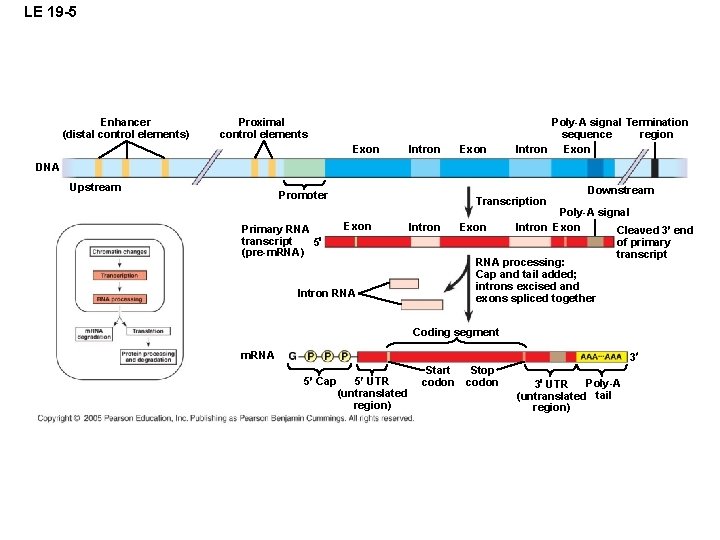
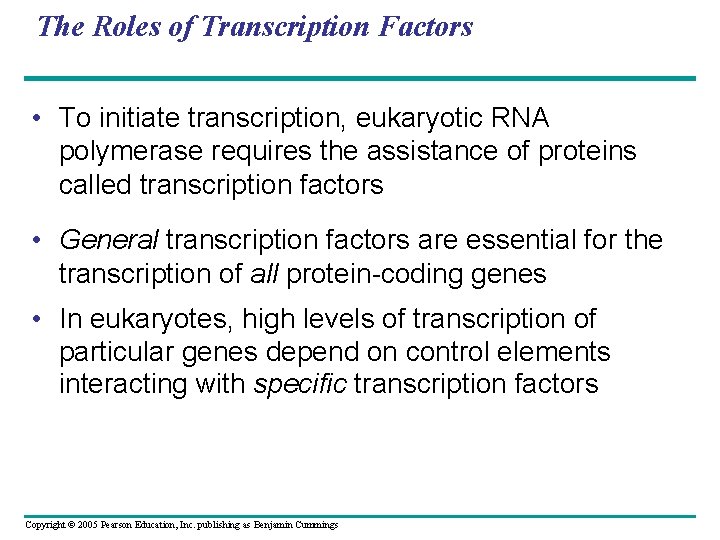
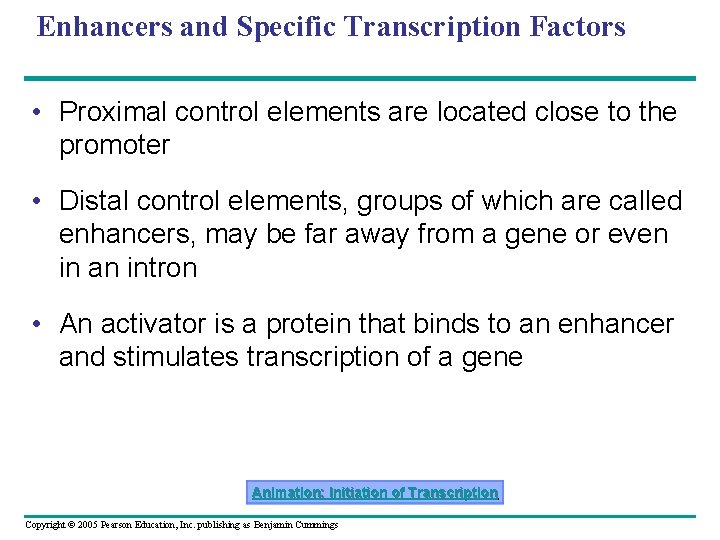
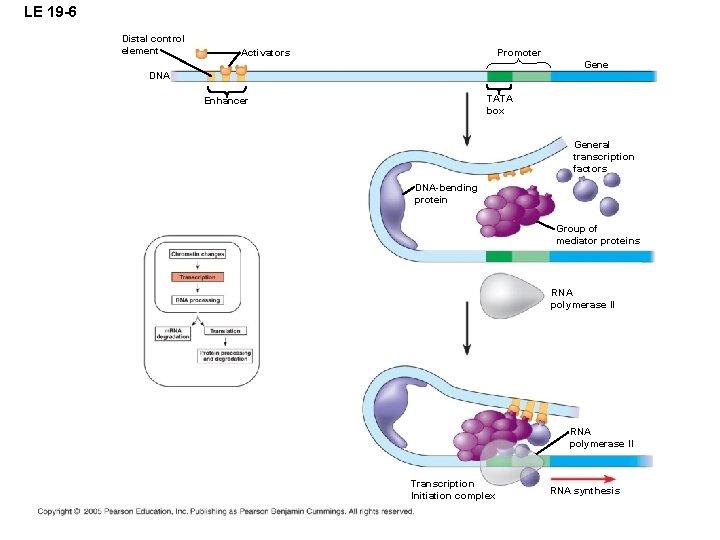
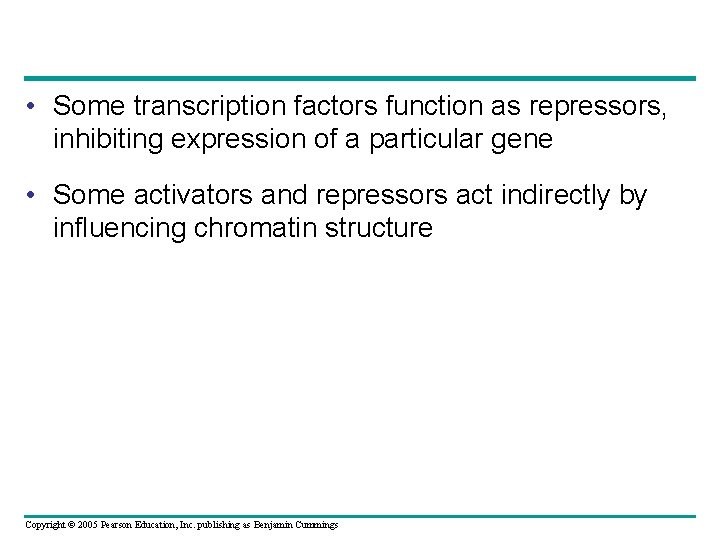
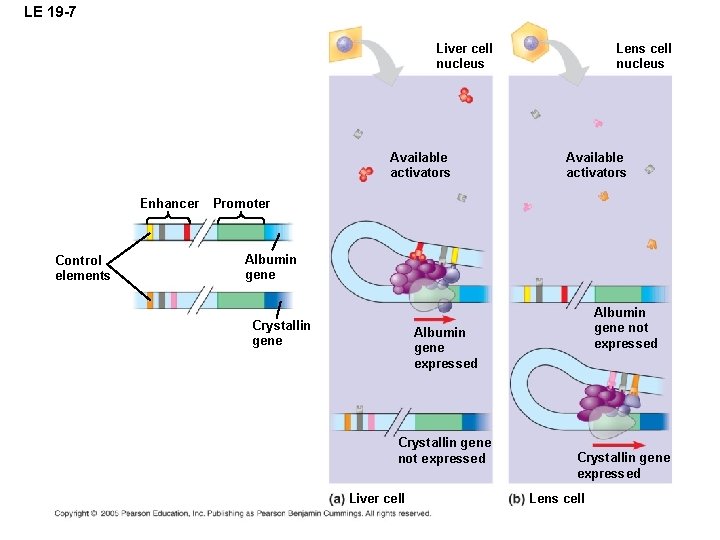
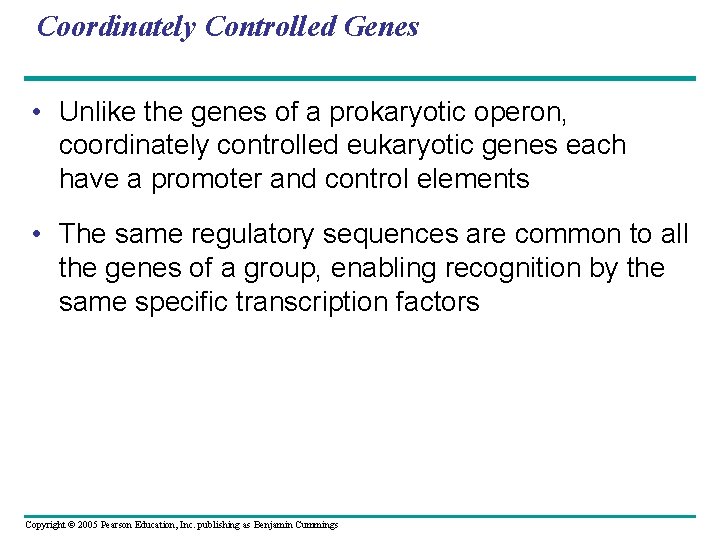
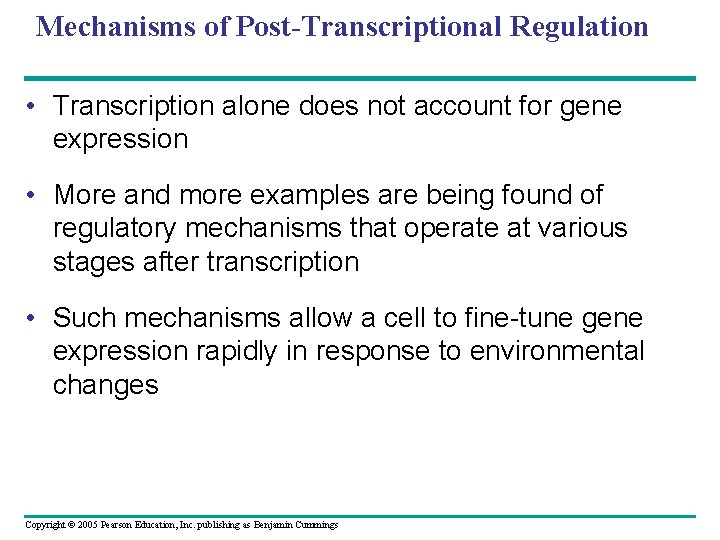
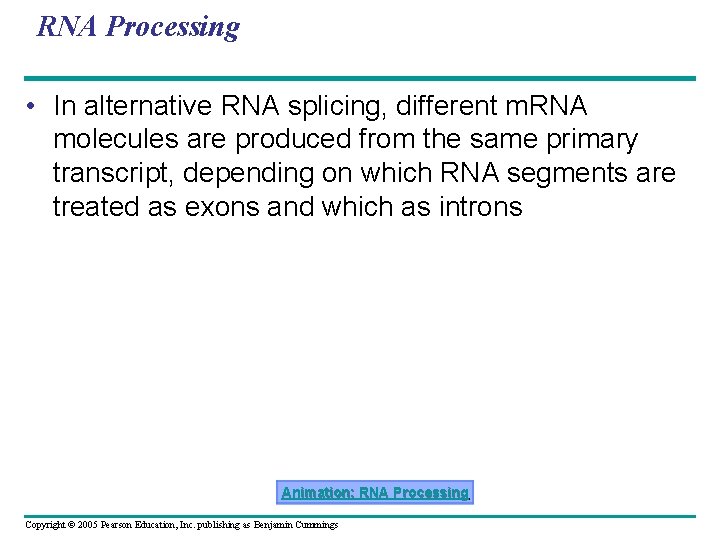
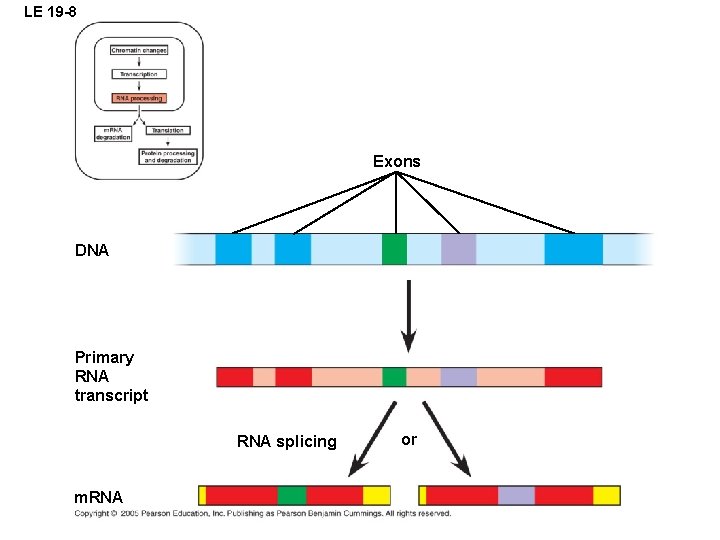
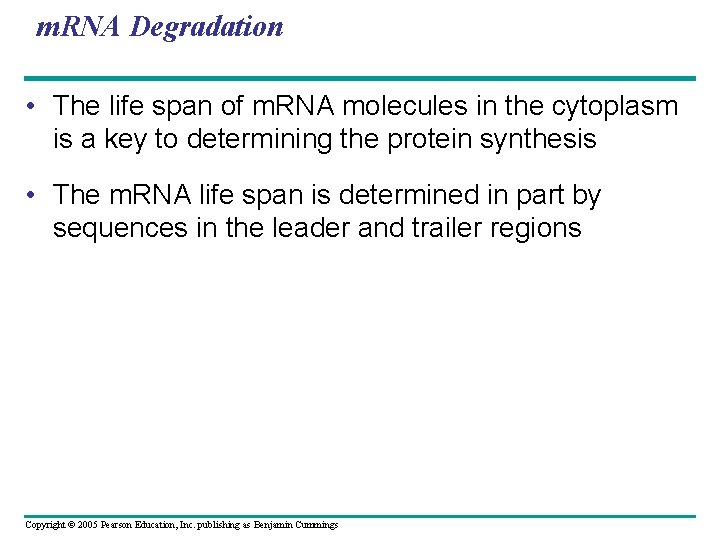
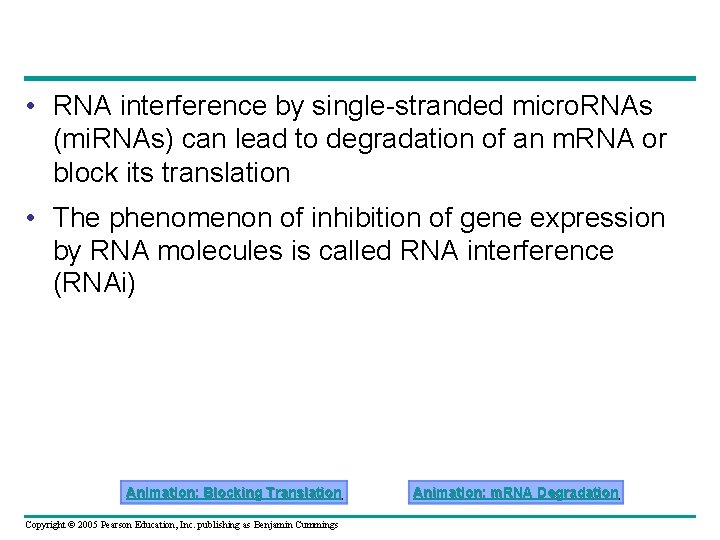
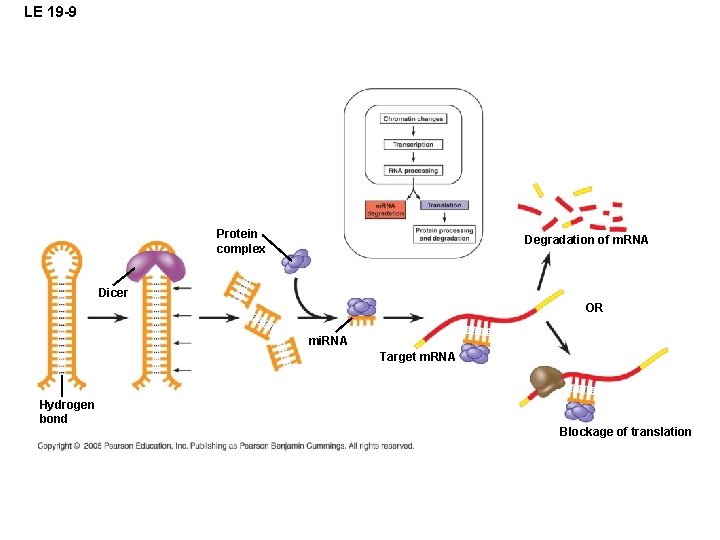
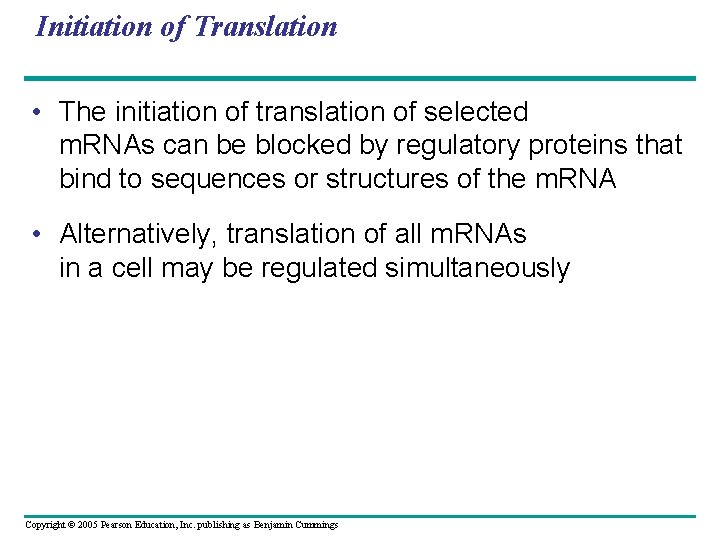
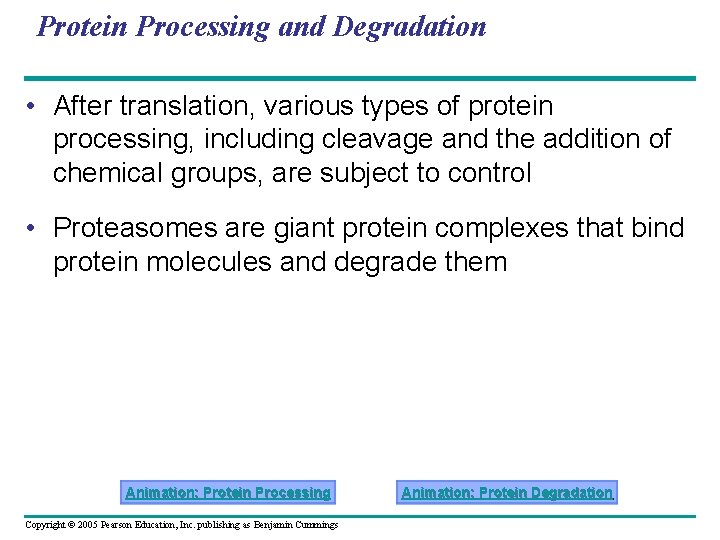
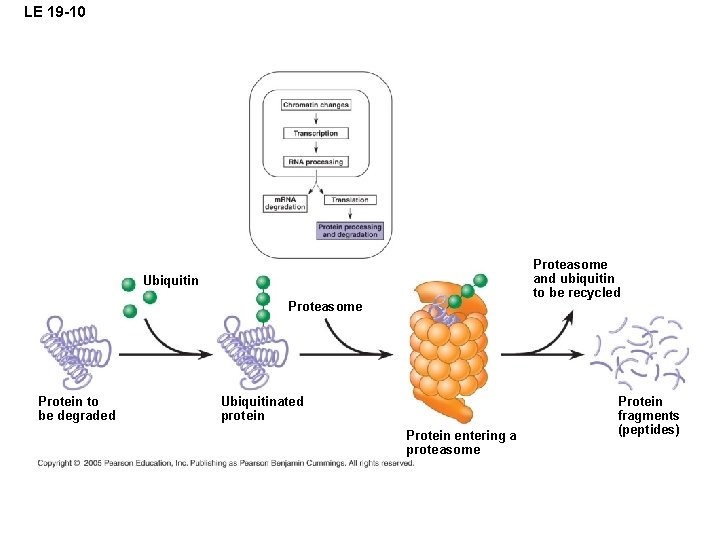
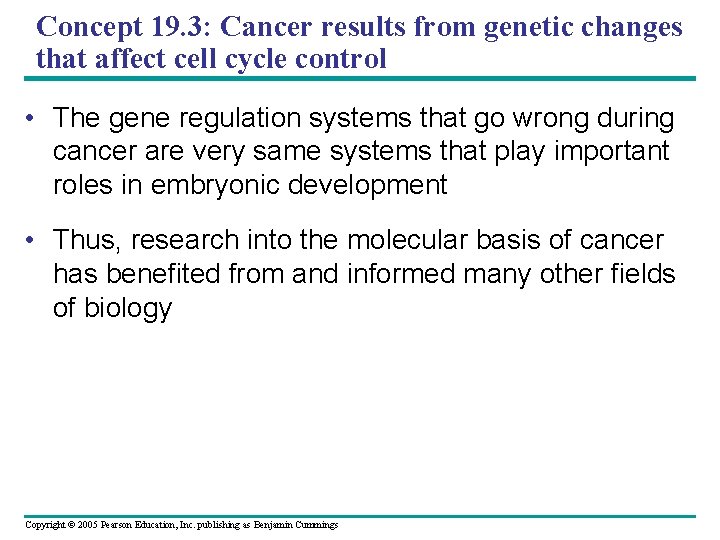
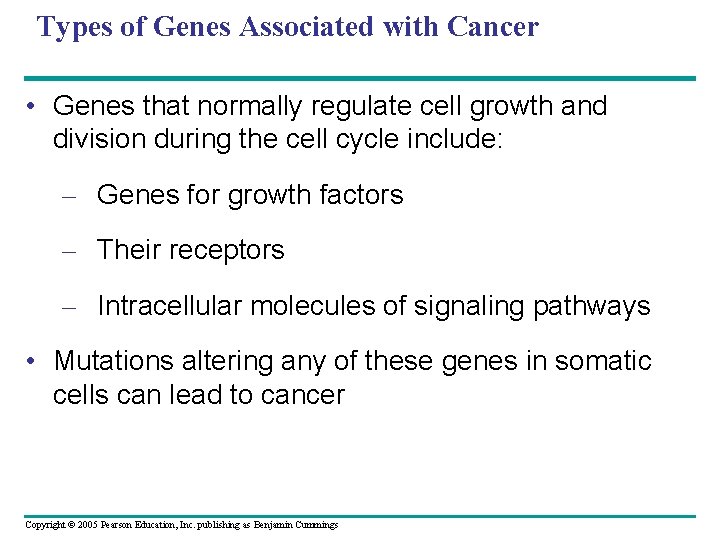
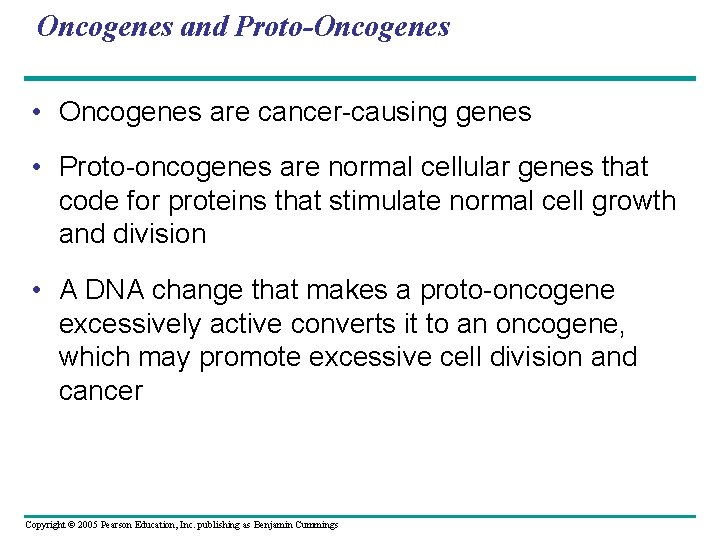
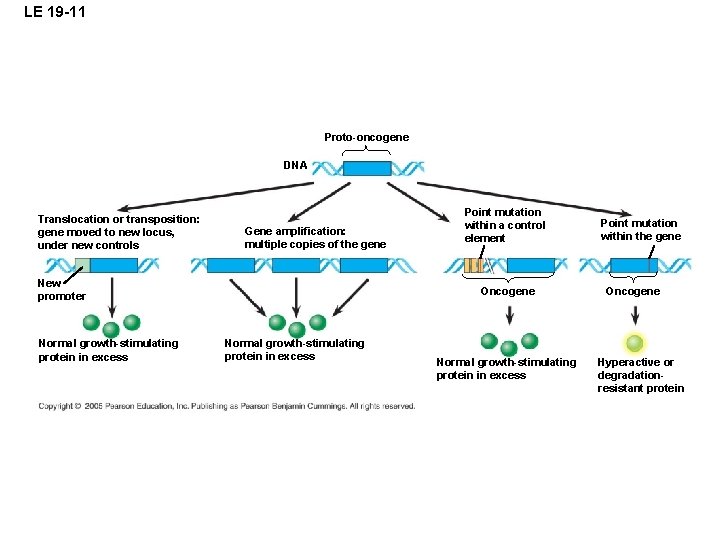
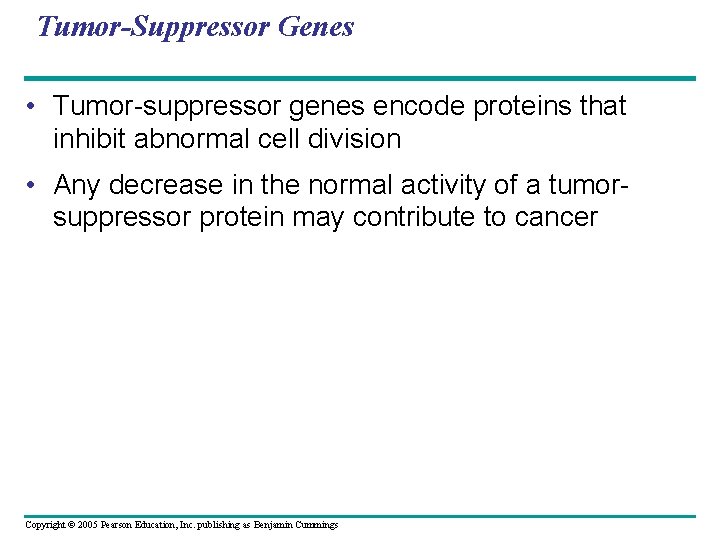
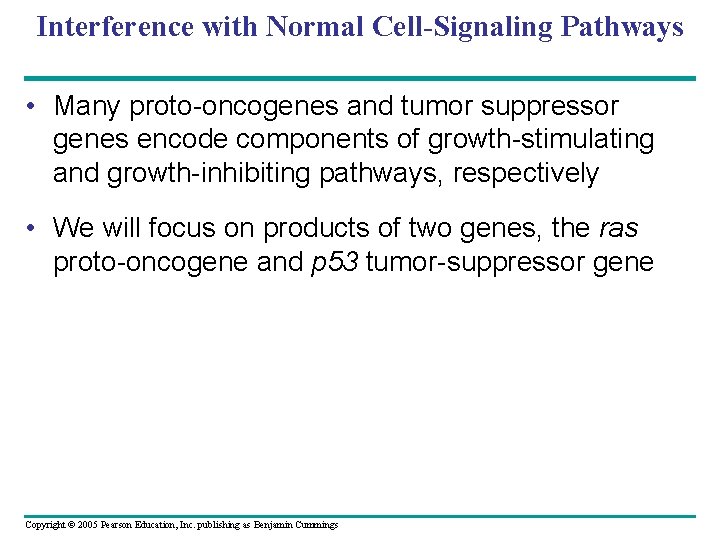
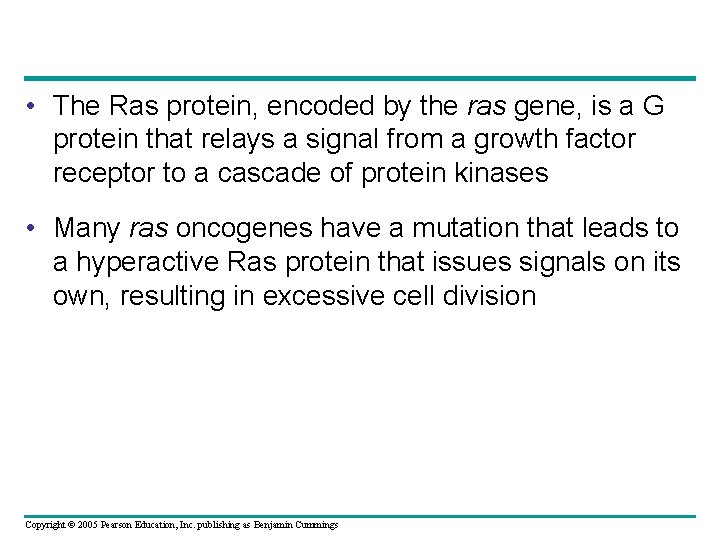
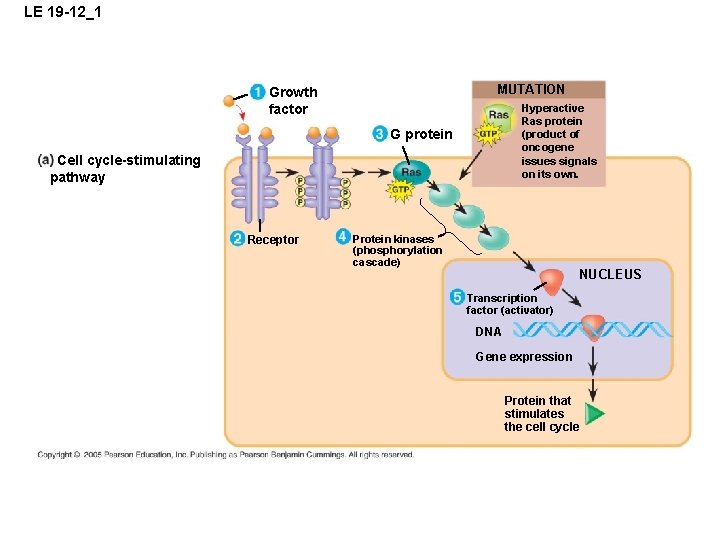
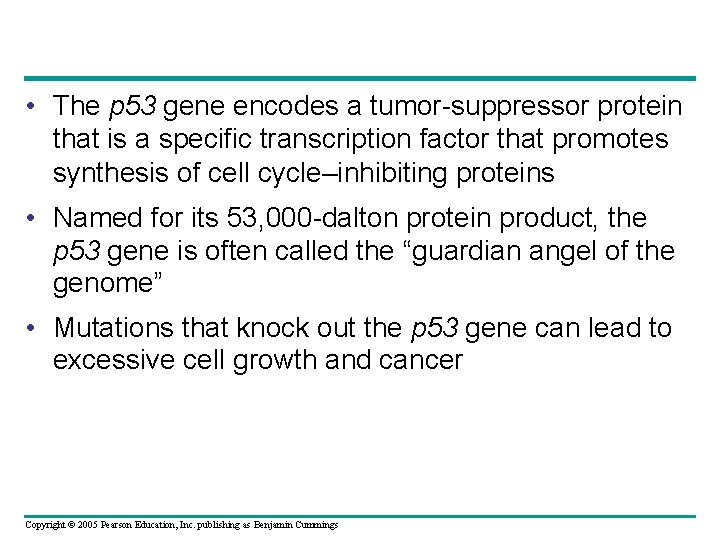
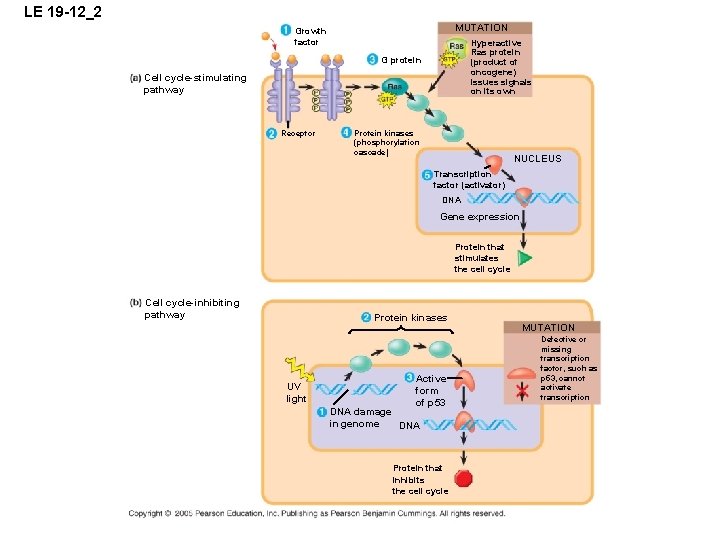
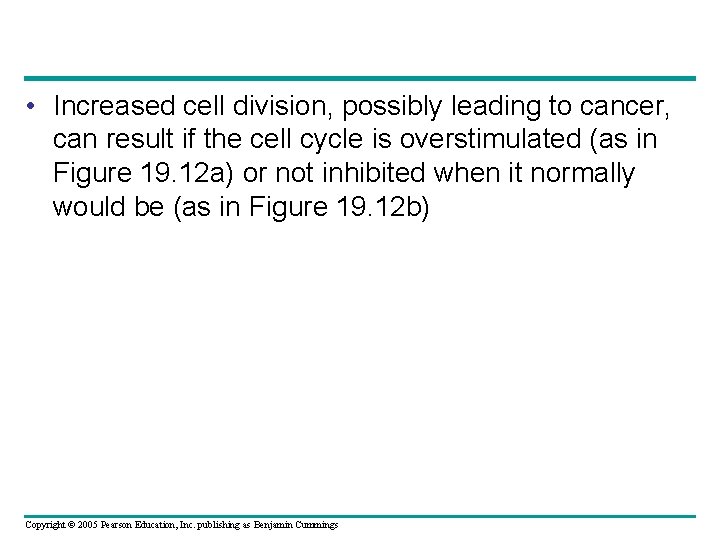
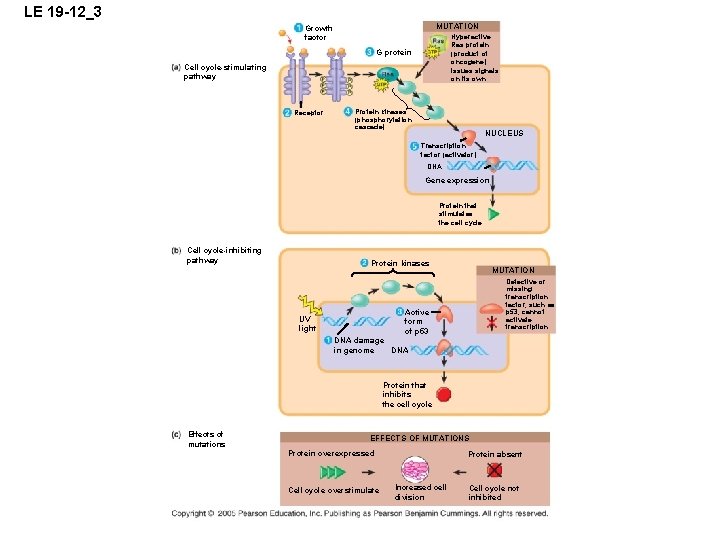
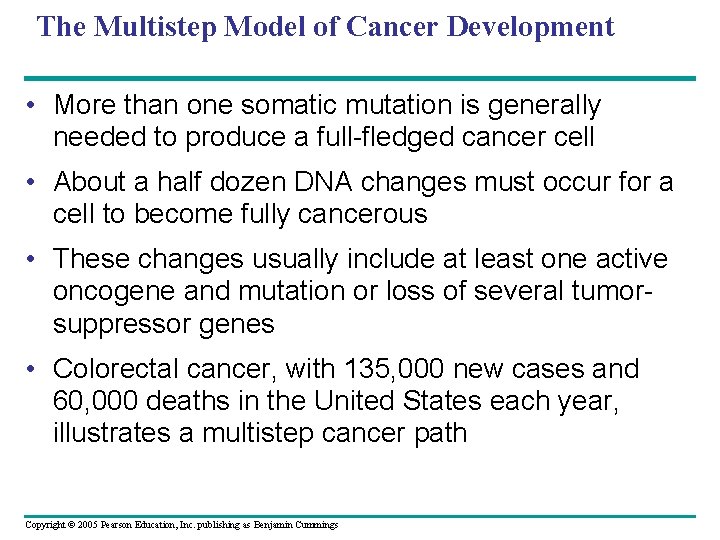
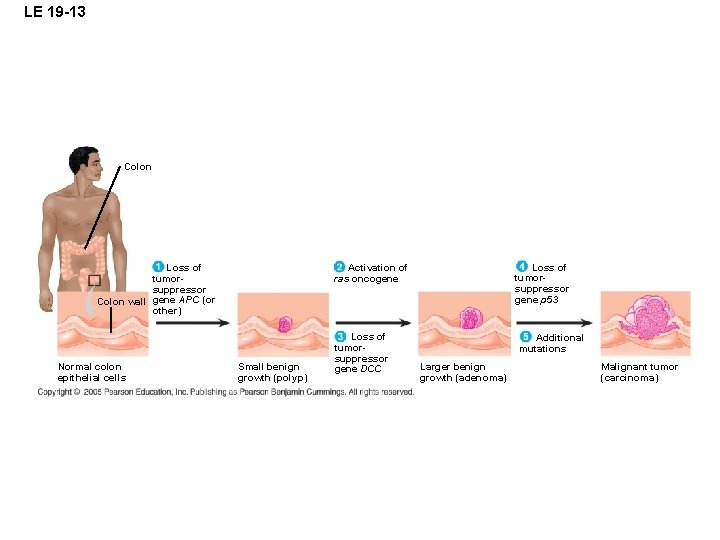
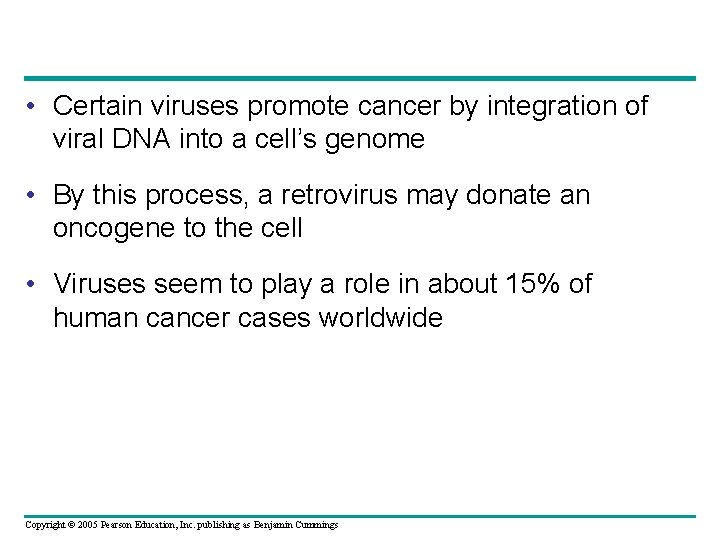
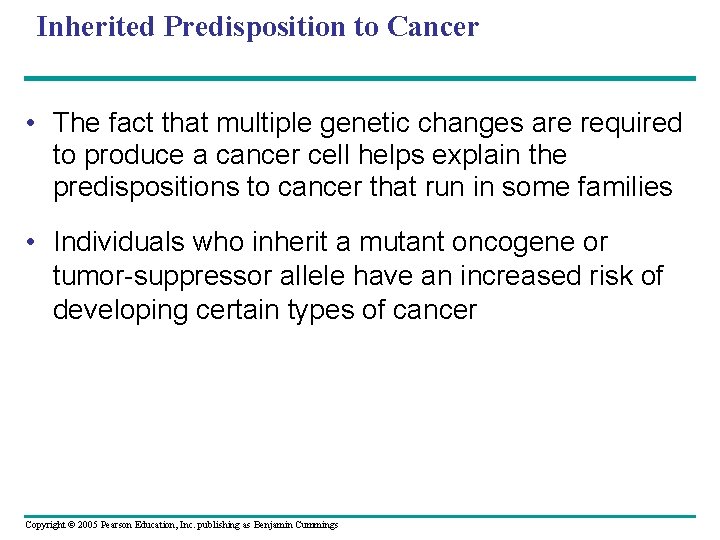
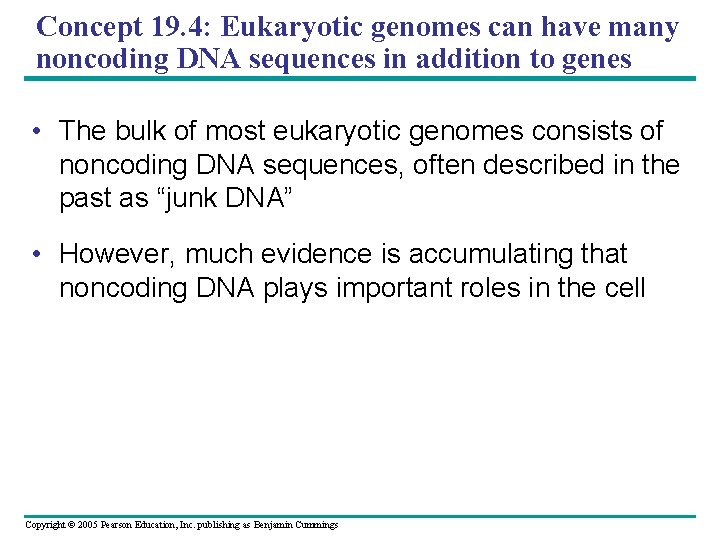
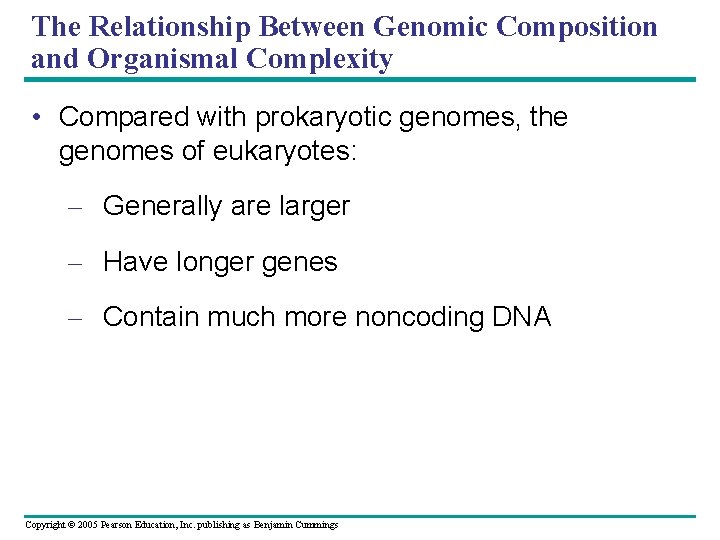
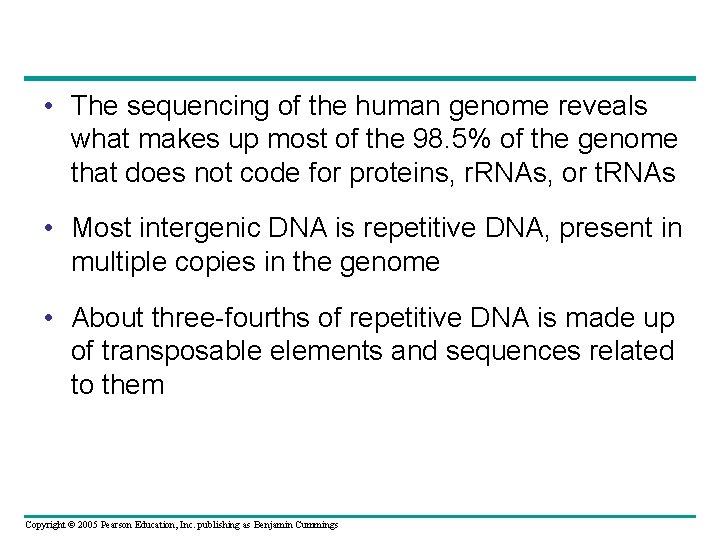
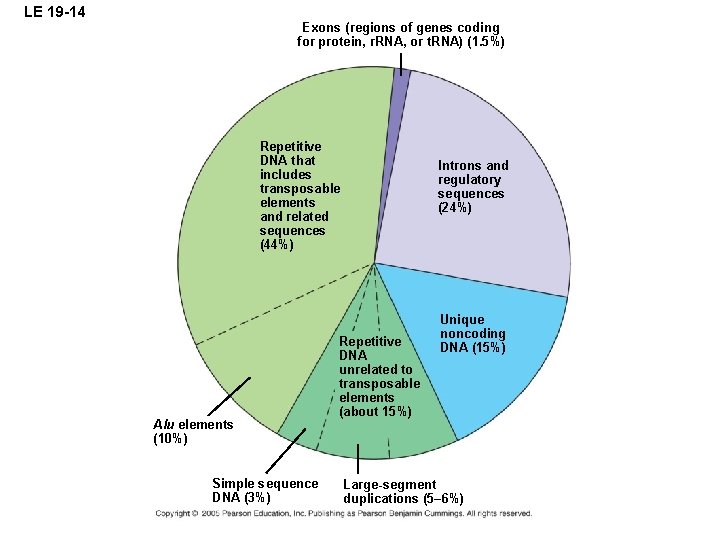
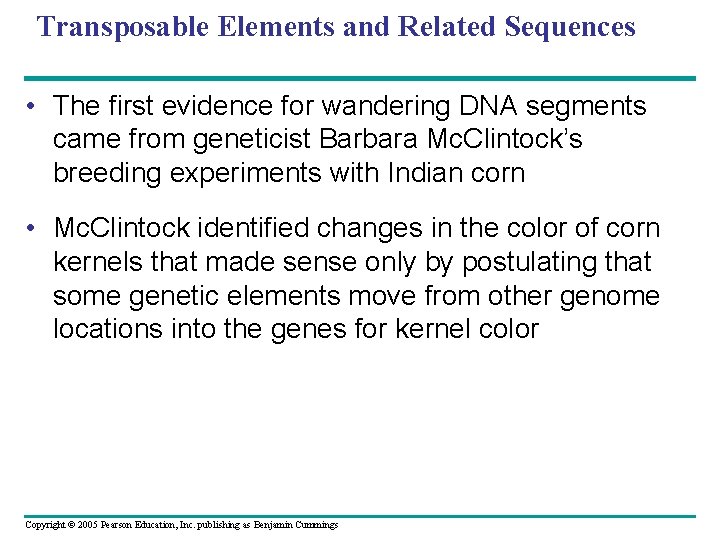
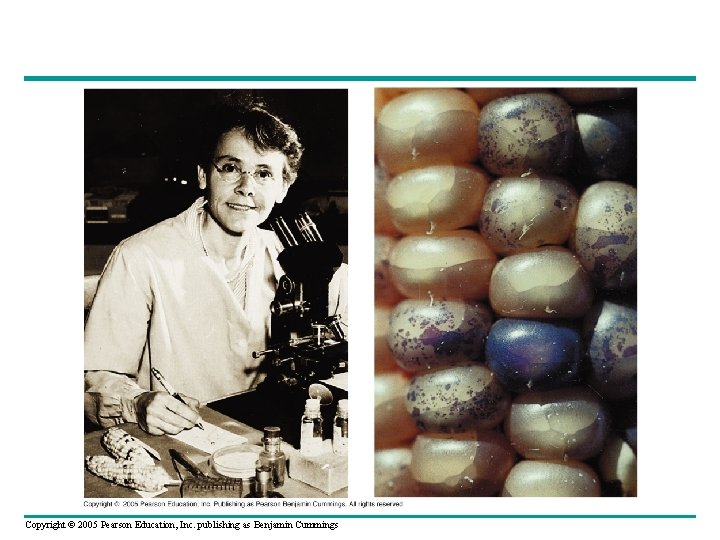
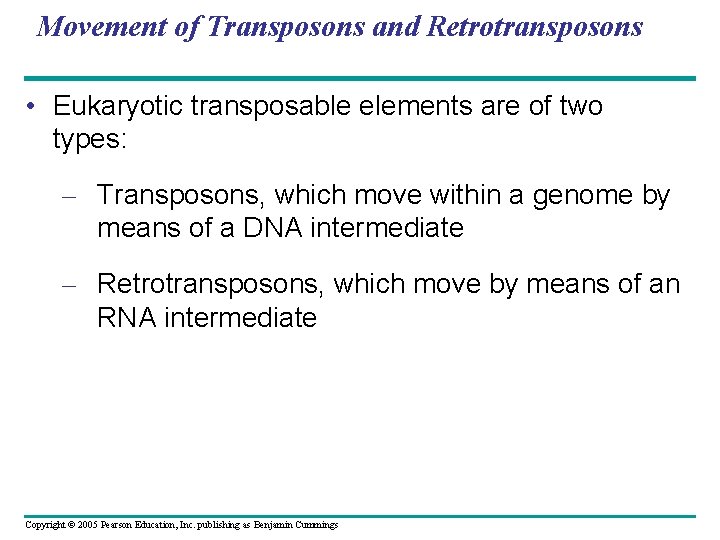
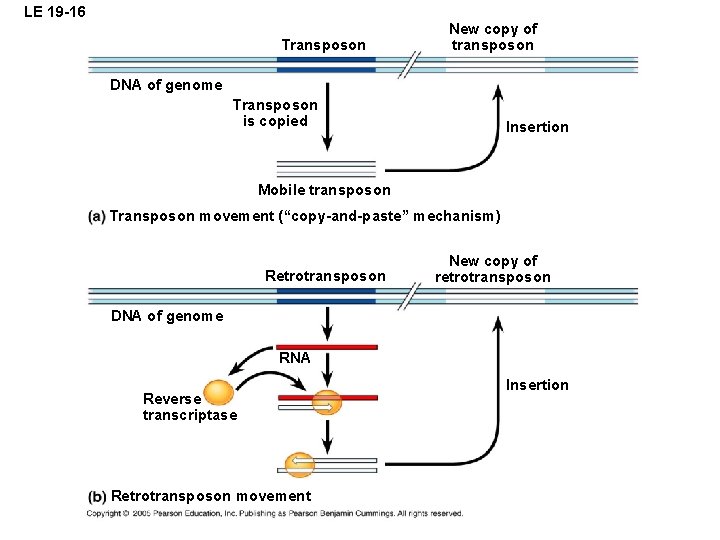
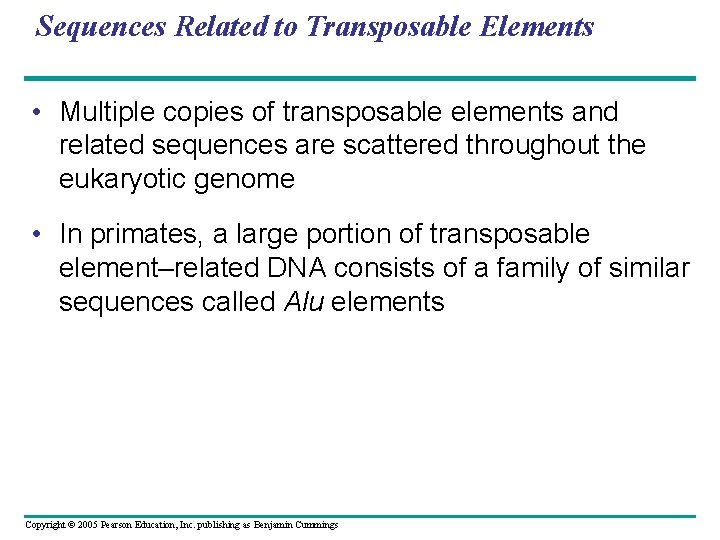
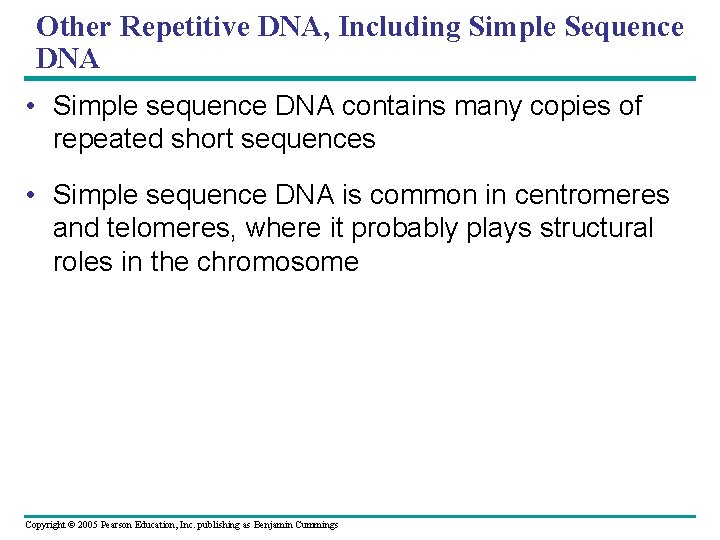
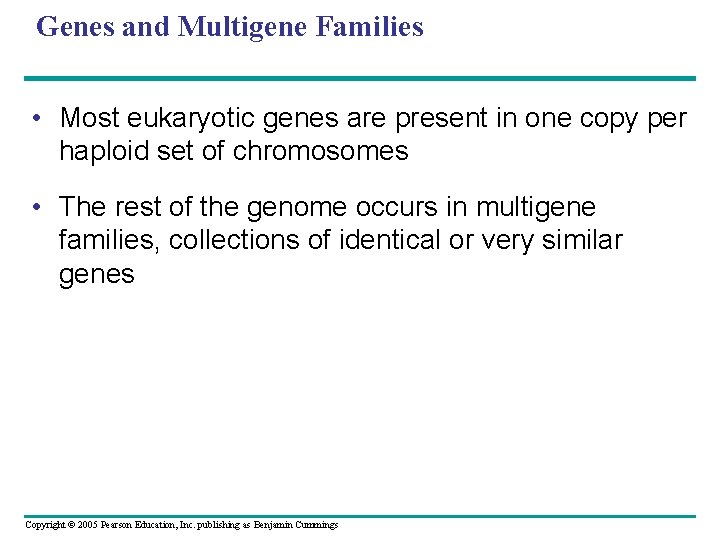
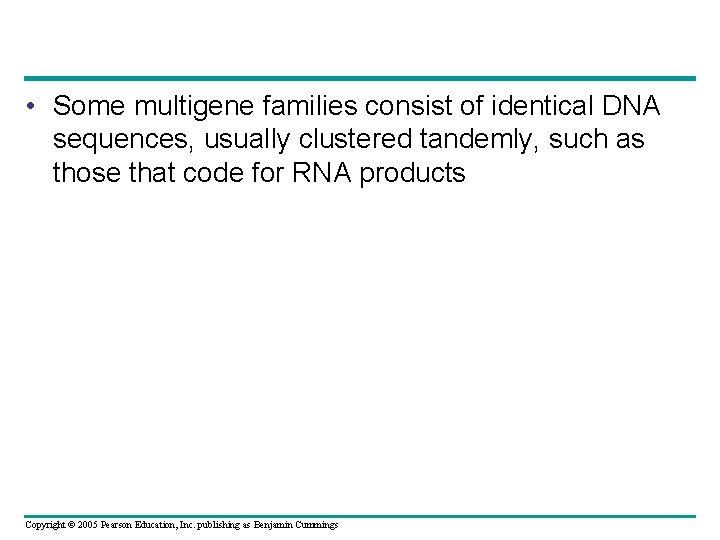
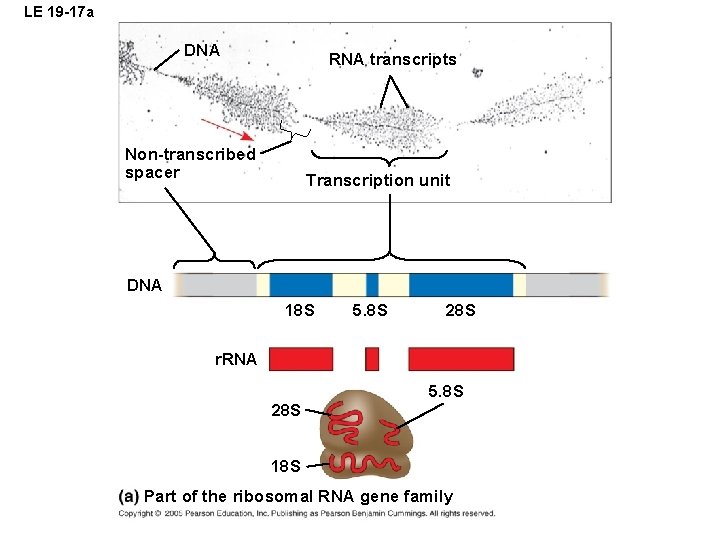
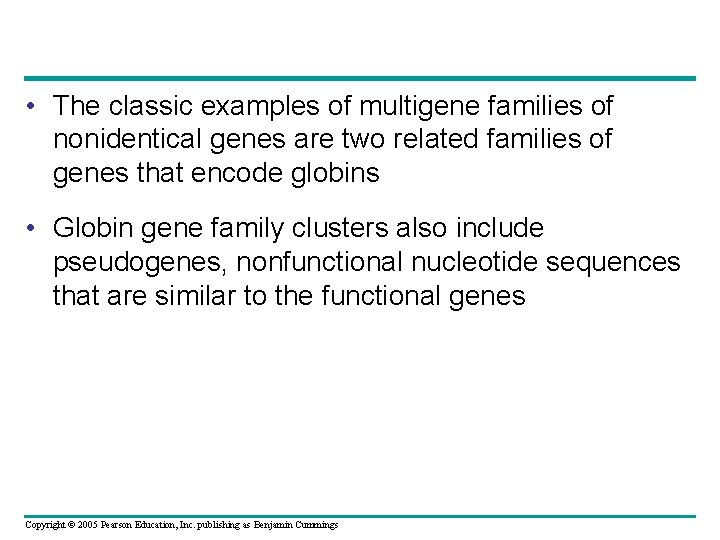
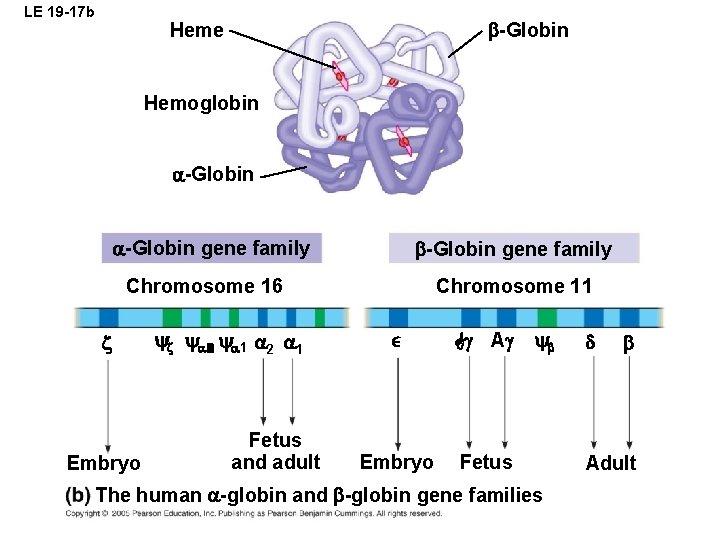
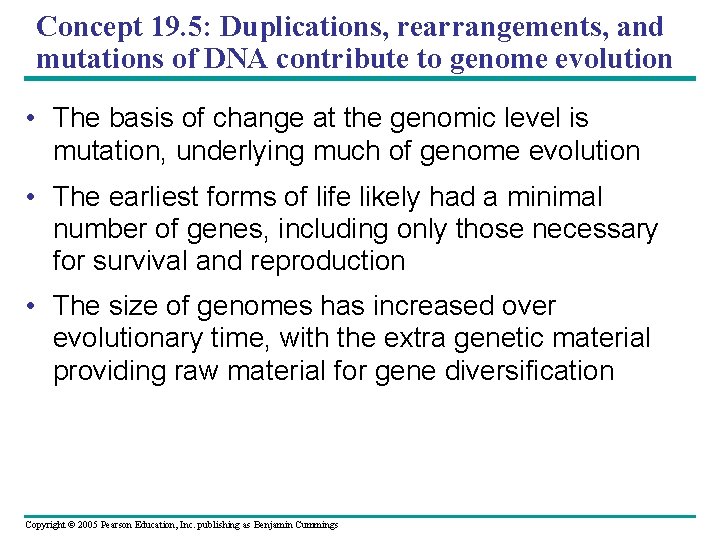
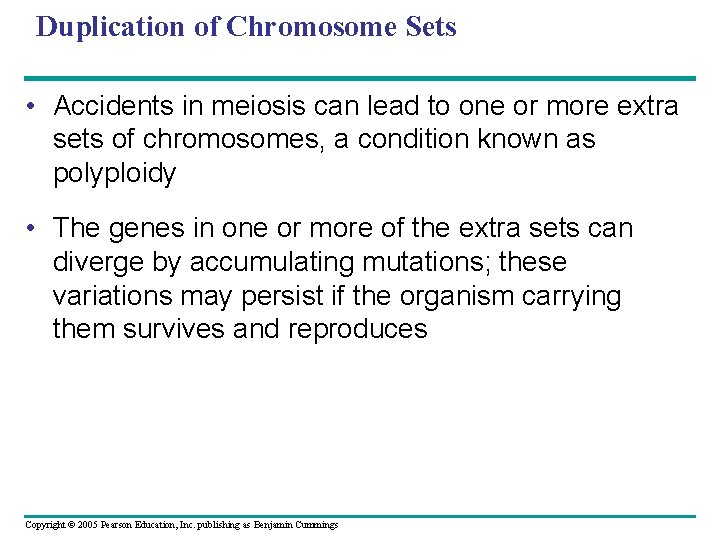
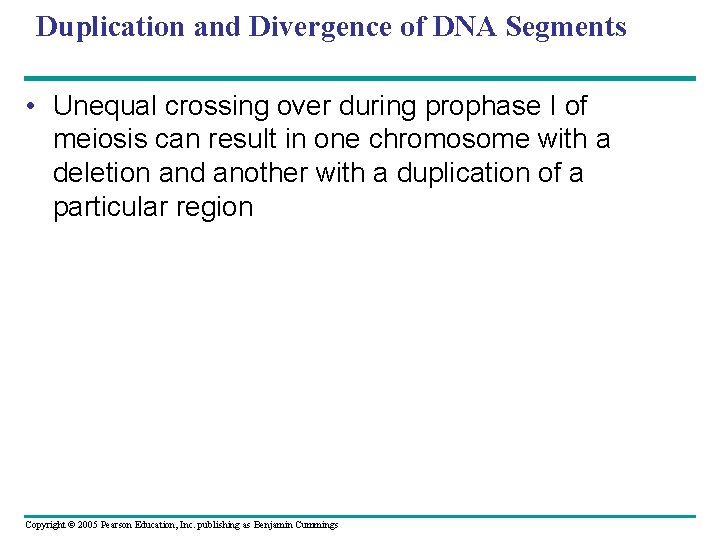
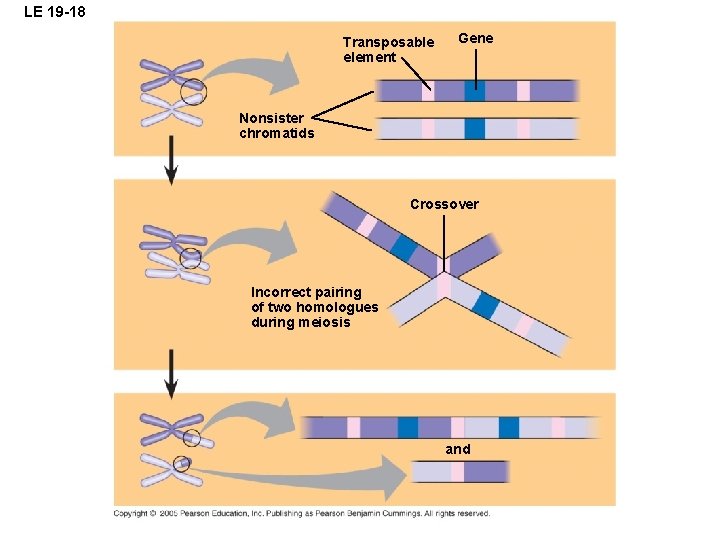
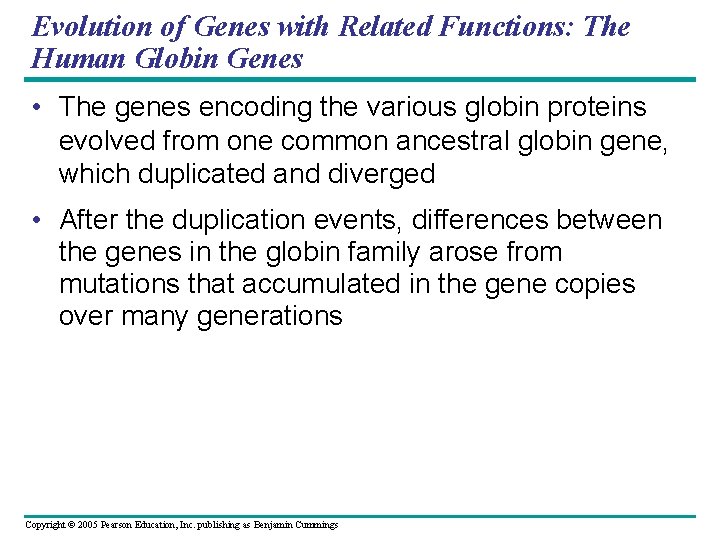
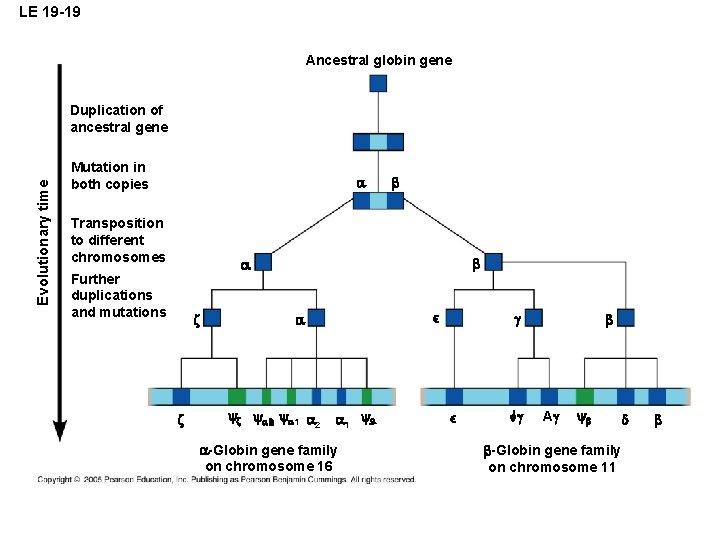
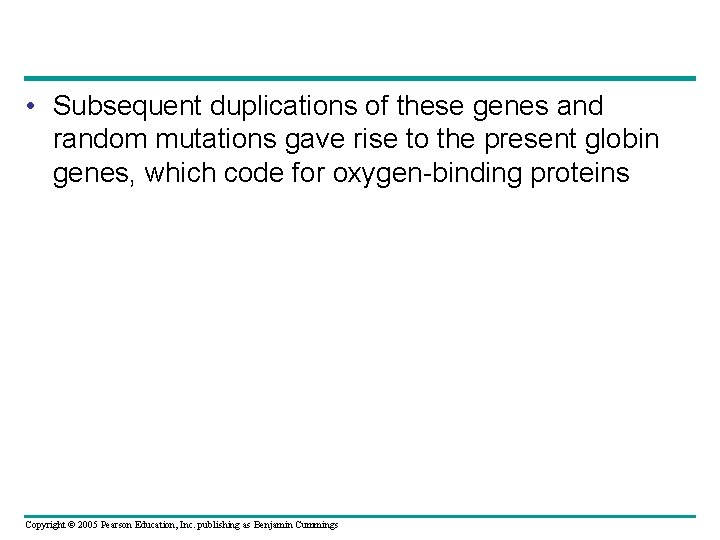
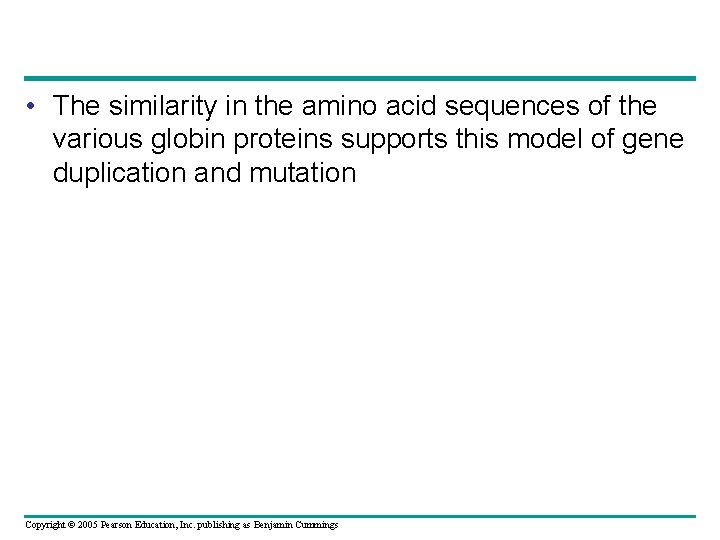
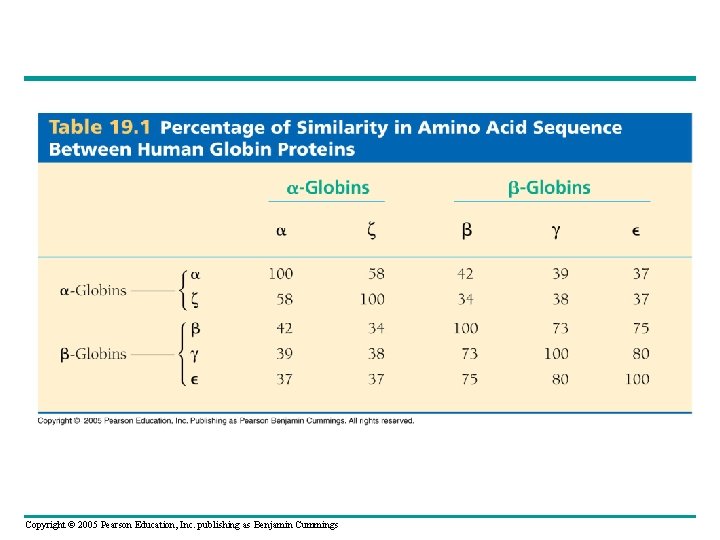
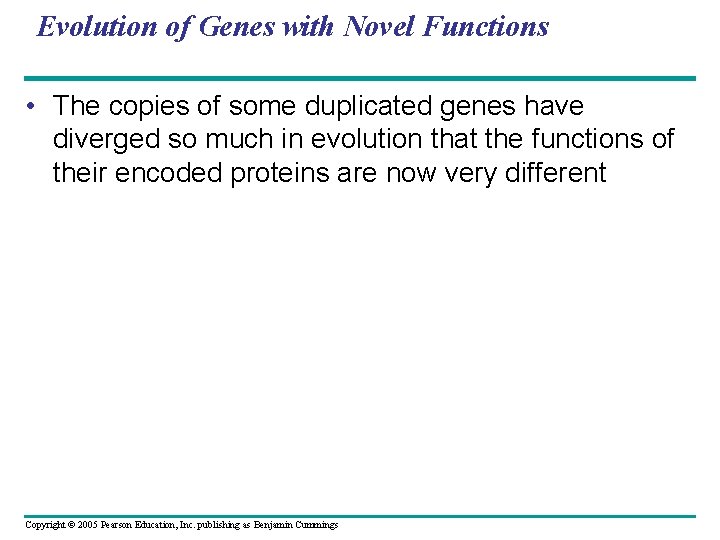
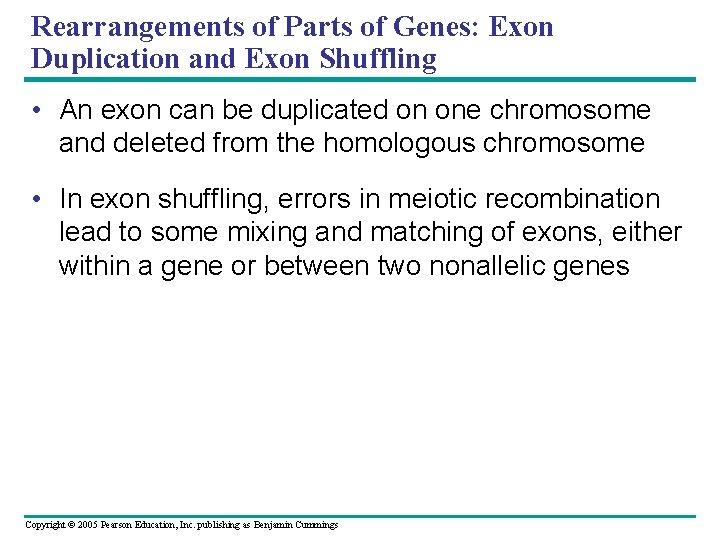
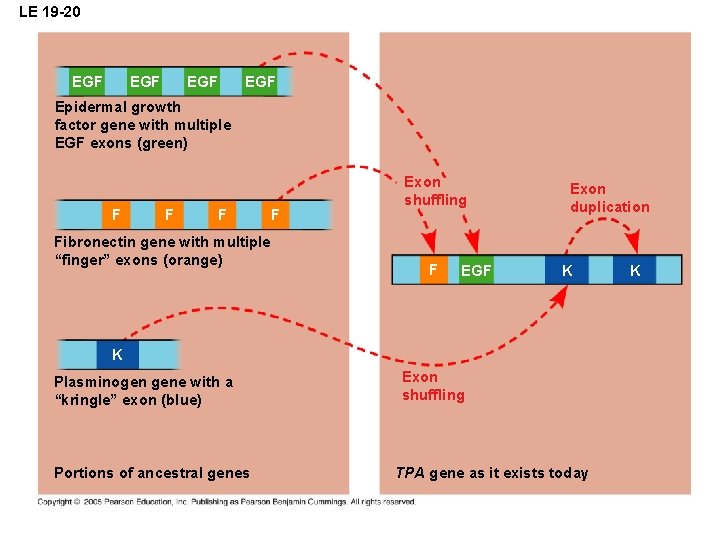
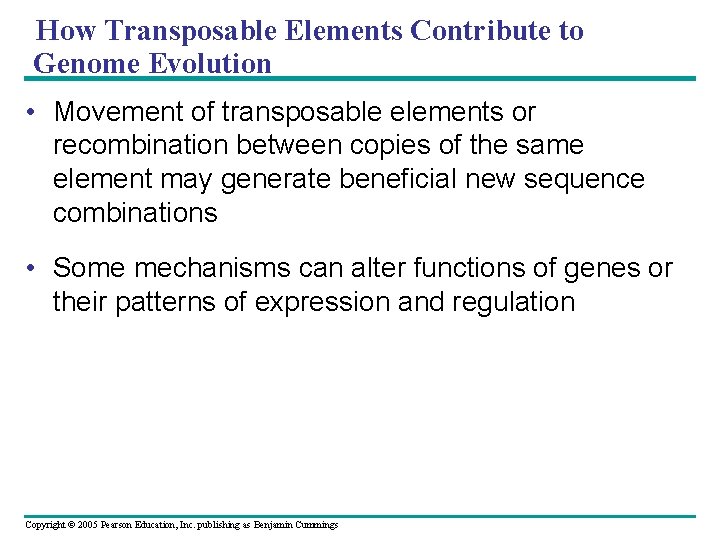
- Slides: 83
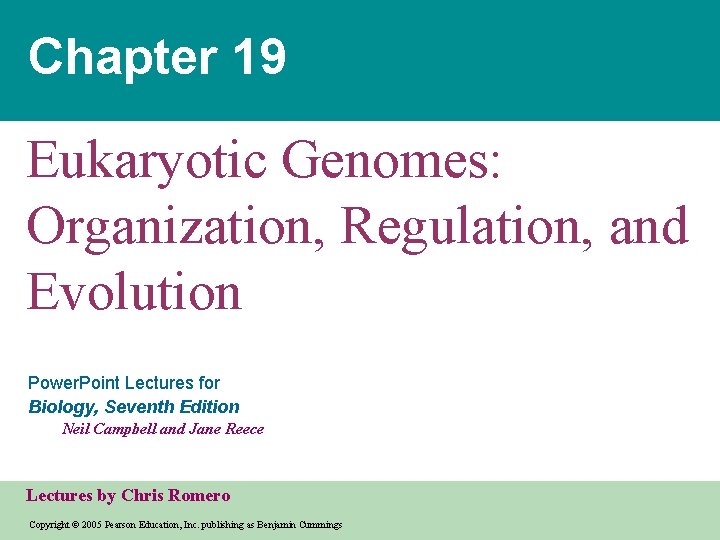
Chapter 19 Eukaryotic Genomes: Organization, Regulation, and Evolution Power. Point Lectures for Biology, Seventh Edition Neil Campbell and Jane Reece Lectures by Chris Romero Copyright © 2005 Pearson Education, Inc. publishing as Benjamin Cummings
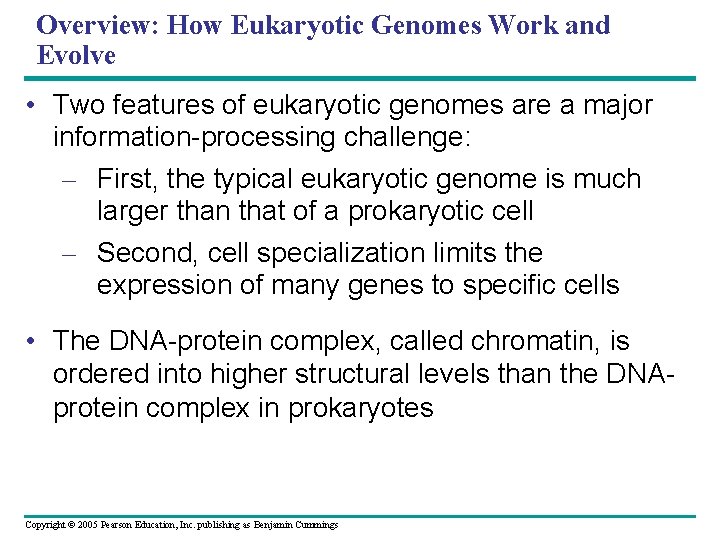
Overview: How Eukaryotic Genomes Work and Evolve • Two features of eukaryotic genomes are a major information-processing challenge: – First, the typical eukaryotic genome is much larger than that of a prokaryotic cell – Second, cell specialization limits the expression of many genes to specific cells • The DNA-protein complex, called chromatin, is ordered into higher structural levels than the DNAprotein complex in prokaryotes Copyright © 2005 Pearson Education, Inc. publishing as Benjamin Cummings
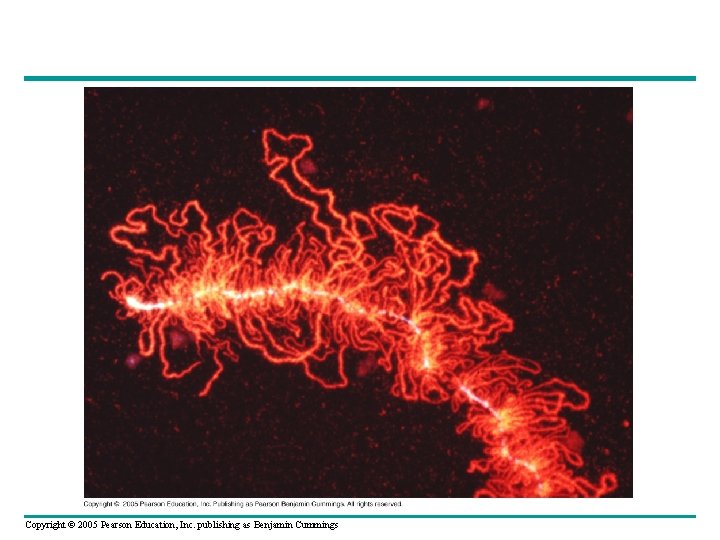
Copyright © 2005 Pearson Education, Inc. publishing as Benjamin Cummings
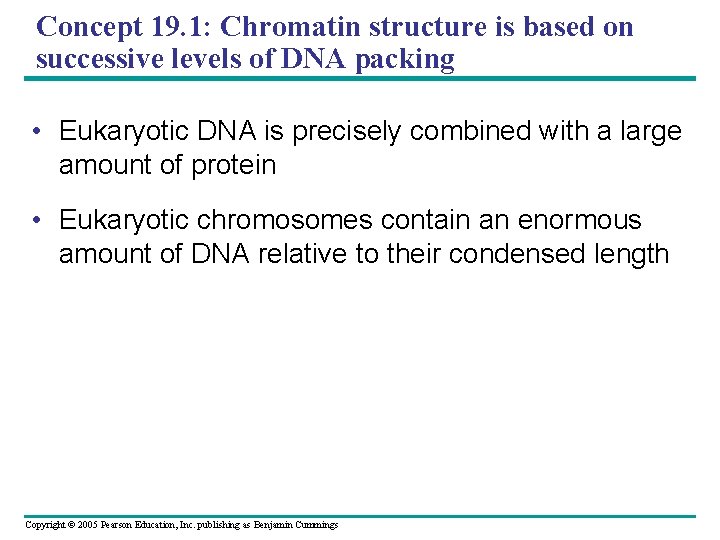
Concept 19. 1: Chromatin structure is based on successive levels of DNA packing • Eukaryotic DNA is precisely combined with a large amount of protein • Eukaryotic chromosomes contain an enormous amount of DNA relative to their condensed length Copyright © 2005 Pearson Education, Inc. publishing as Benjamin Cummings
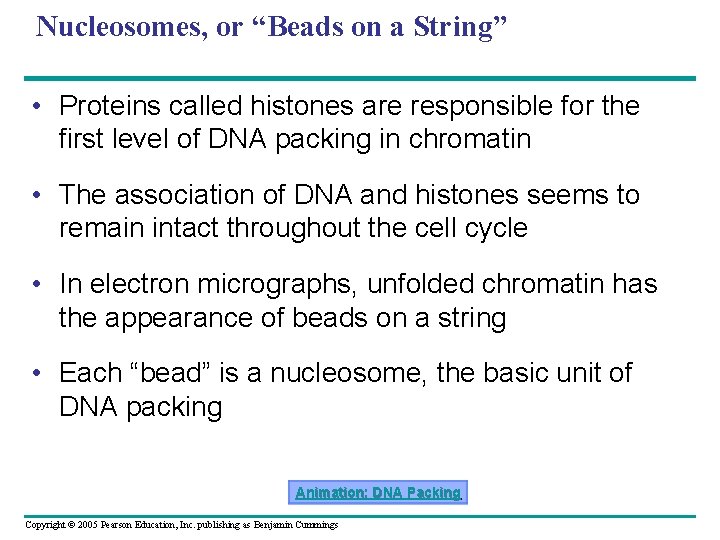
Nucleosomes, or “Beads on a String” • Proteins called histones are responsible for the first level of DNA packing in chromatin • The association of DNA and histones seems to remain intact throughout the cell cycle • In electron micrographs, unfolded chromatin has the appearance of beads on a string • Each “bead” is a nucleosome, the basic unit of DNA packing Animation: DNA Packing Copyright © 2005 Pearson Education, Inc. publishing as Benjamin Cummings
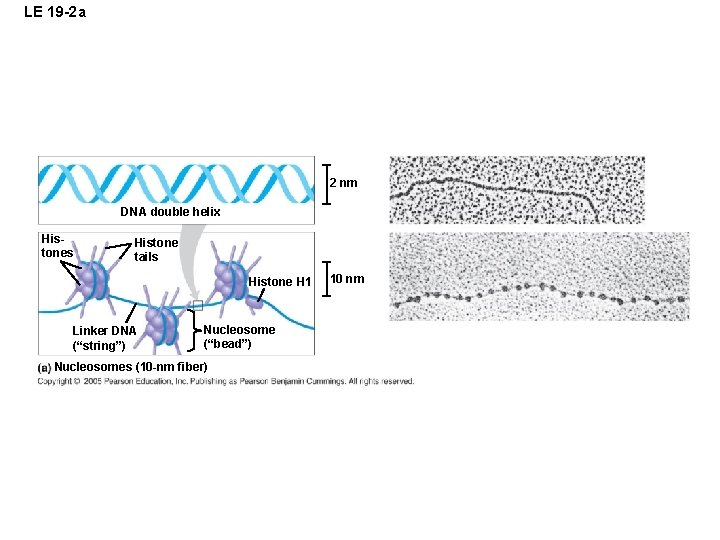
LE 19 -2 a 2 nm DNA double helix Histones Histone tails Histone H 1 Linker DNA (“string”) Nucleosome (“bead”) Nucleosomes (10 -nm fiber) 10 nm
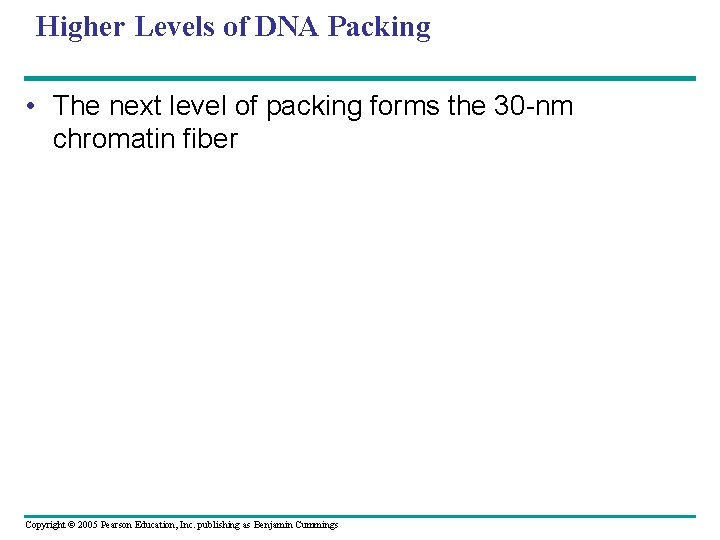
Higher Levels of DNA Packing • The next level of packing forms the 30 -nm chromatin fiber Copyright © 2005 Pearson Education, Inc. publishing as Benjamin Cummings
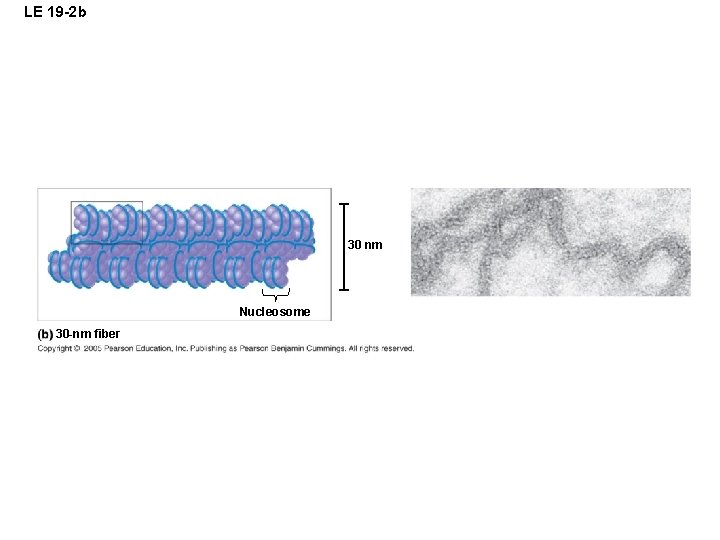
LE 19 -2 b 30 nm Nucleosome 30 -nm fiber
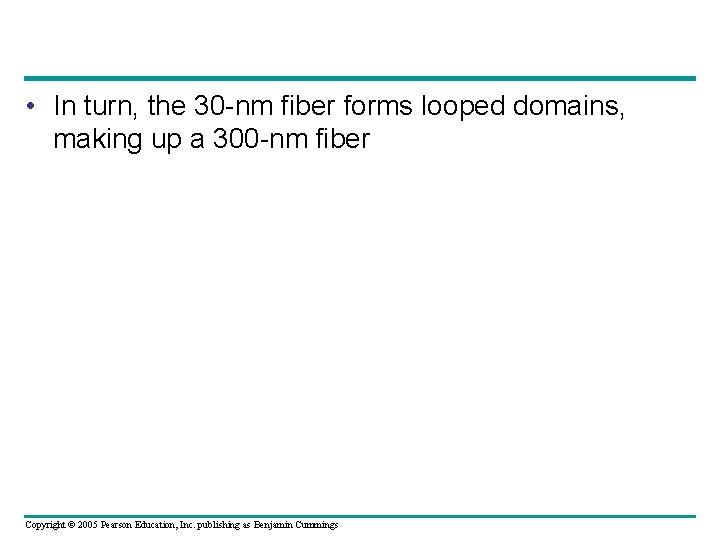
• In turn, the 30 -nm fiber forms looped domains, making up a 300 -nm fiber Copyright © 2005 Pearson Education, Inc. publishing as Benjamin Cummings
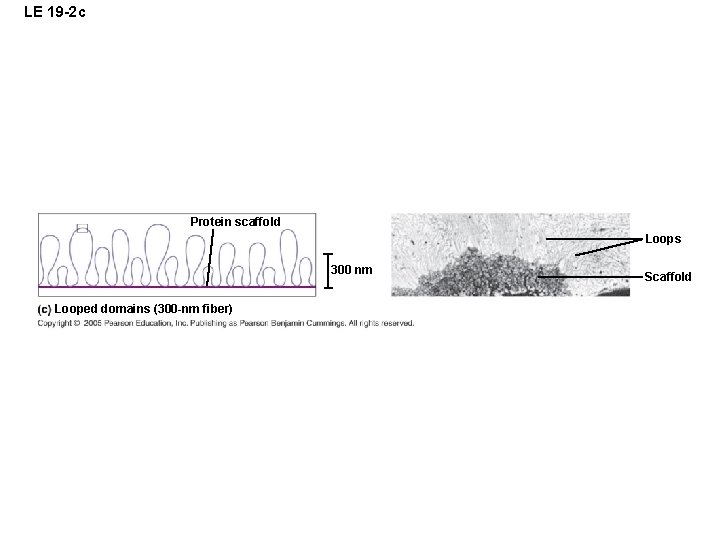
LE 19 -2 c Protein scaffold Loops 300 nm Looped domains (300 -nm fiber) Scaffold
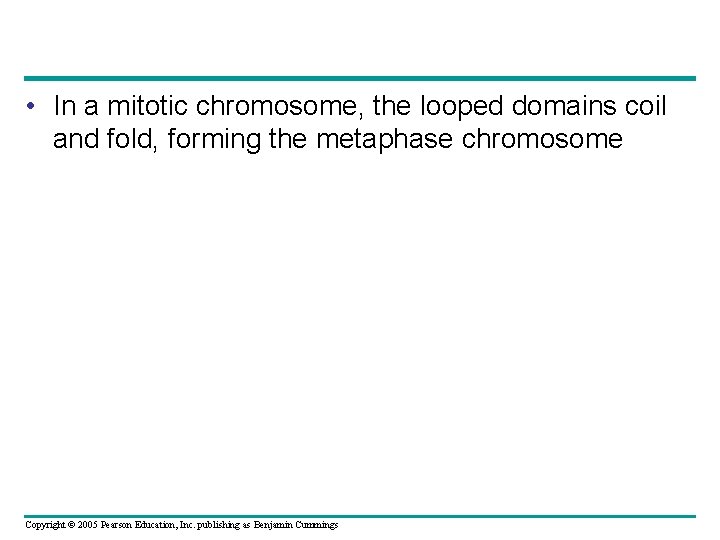
• In a mitotic chromosome, the looped domains coil and fold, forming the metaphase chromosome Copyright © 2005 Pearson Education, Inc. publishing as Benjamin Cummings
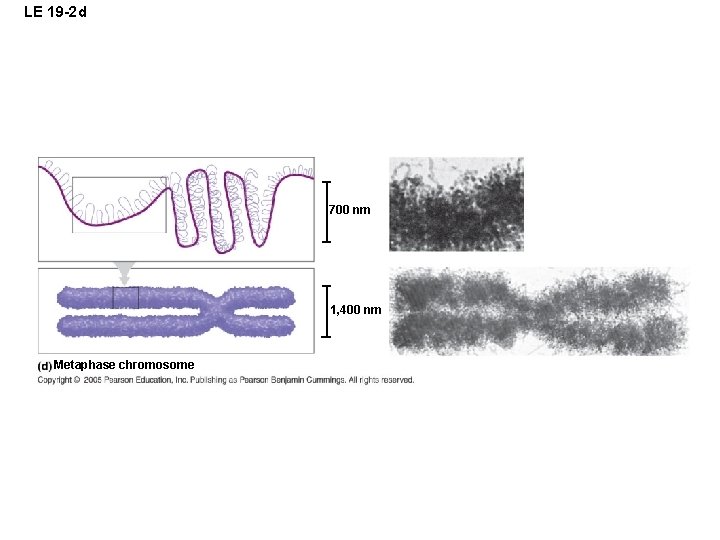
LE 19 -2 d 700 nm 1, 400 nm Metaphase chromosome
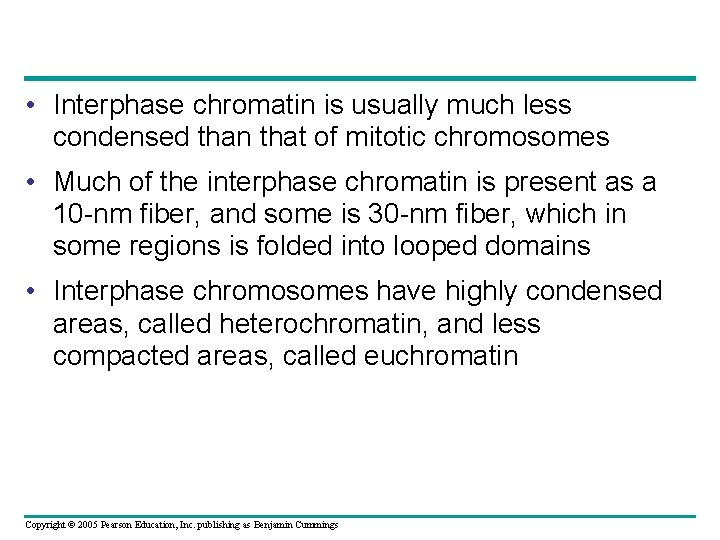
• Interphase chromatin is usually much less condensed than that of mitotic chromosomes • Much of the interphase chromatin is present as a 10 -nm fiber, and some is 30 -nm fiber, which in some regions is folded into looped domains • Interphase chromosomes have highly condensed areas, called heterochromatin, and less compacted areas, called euchromatin Copyright © 2005 Pearson Education, Inc. publishing as Benjamin Cummings
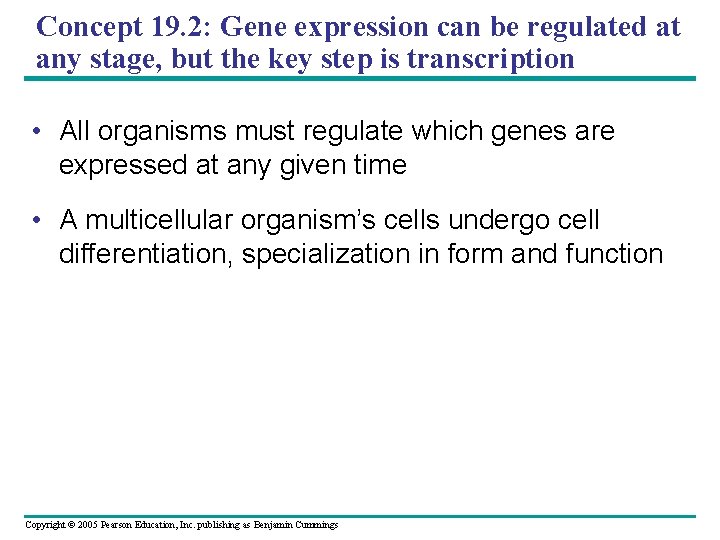
Concept 19. 2: Gene expression can be regulated at any stage, but the key step is transcription • All organisms must regulate which genes are expressed at any given time • A multicellular organism’s cells undergo cell differentiation, specialization in form and function Copyright © 2005 Pearson Education, Inc. publishing as Benjamin Cummings
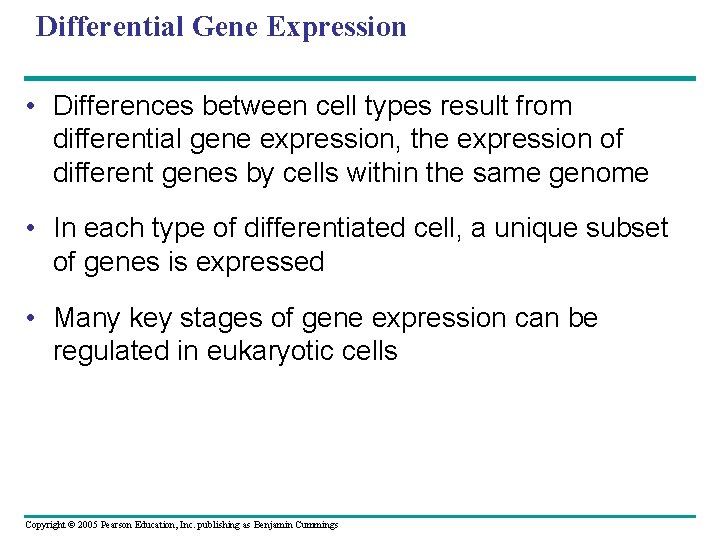
Differential Gene Expression • Differences between cell types result from differential gene expression, the expression of different genes by cells within the same genome • In each type of differentiated cell, a unique subset of genes is expressed • Many key stages of gene expression can be regulated in eukaryotic cells Copyright © 2005 Pearson Education, Inc. publishing as Benjamin Cummings
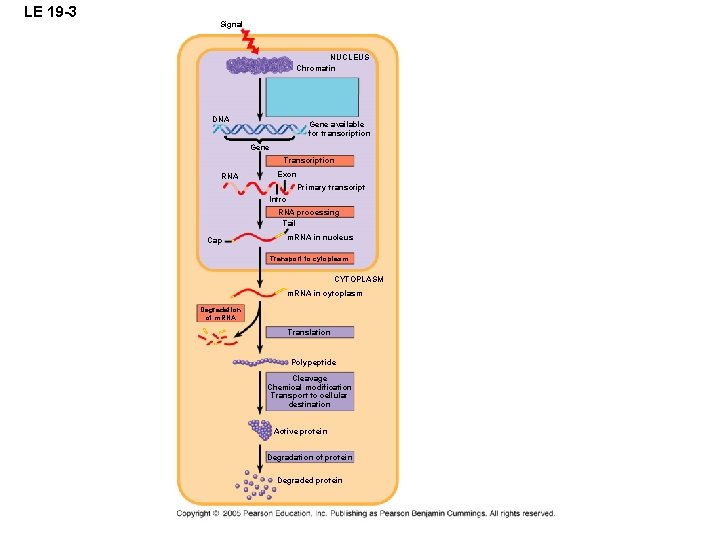
LE 19 -3 Signal NUCLEUS Chromatin DNA Gene available for transcription Gene Transcription RNA Exon Primary transcript Intro RNA processing Tail Cap m. RNA in nucleus Transport to cytoplasm CYTOPLASM m. RNA in cytoplasm Degradation of m. RNA Translation Polypeptide Cleavage Chemical modification Transport to cellular destination Active protein Degradation of protein Degraded protein
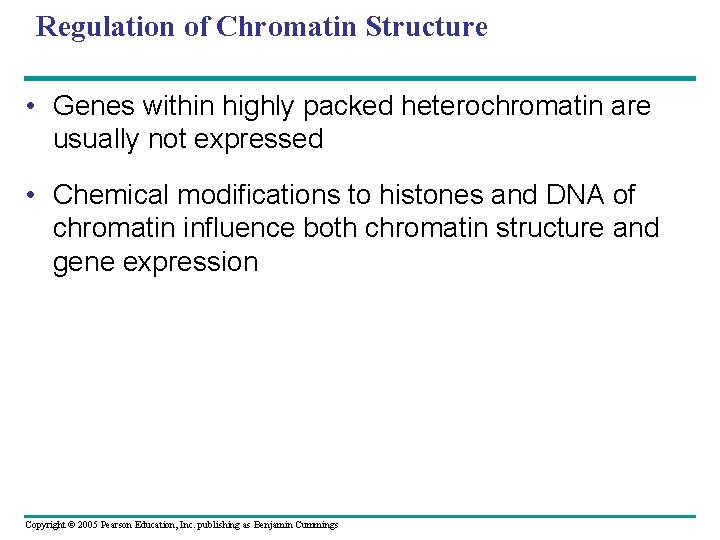
Regulation of Chromatin Structure • Genes within highly packed heterochromatin are usually not expressed • Chemical modifications to histones and DNA of chromatin influence both chromatin structure and gene expression Copyright © 2005 Pearson Education, Inc. publishing as Benjamin Cummings
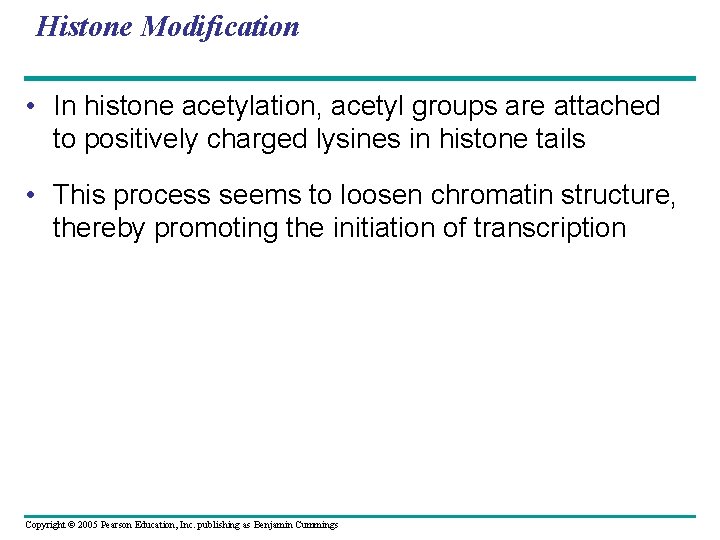
Histone Modification • In histone acetylation, acetyl groups are attached to positively charged lysines in histone tails • This process seems to loosen chromatin structure, thereby promoting the initiation of transcription Copyright © 2005 Pearson Education, Inc. publishing as Benjamin Cummings
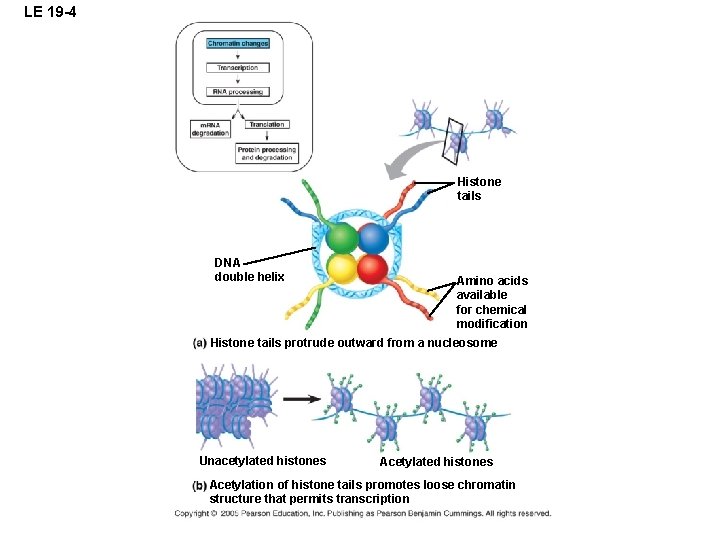
LE 19 -4 Histone tails DNA double helix Amino acids available for chemical modification Histone tails protrude outward from a nucleosome Unacetylated histones Acetylation of histone tails promotes loose chromatin structure that permits transcription
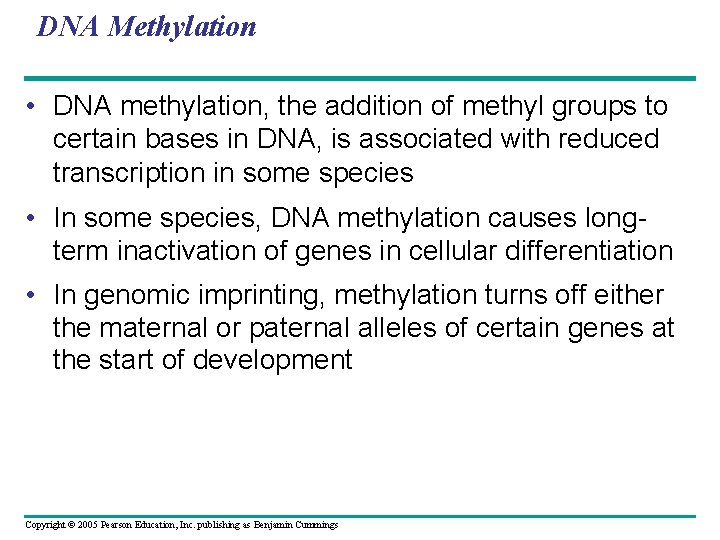
DNA Methylation • DNA methylation, the addition of methyl groups to certain bases in DNA, is associated with reduced transcription in some species • In some species, DNA methylation causes longterm inactivation of genes in cellular differentiation • In genomic imprinting, methylation turns off either the maternal or paternal alleles of certain genes at the start of development Copyright © 2005 Pearson Education, Inc. publishing as Benjamin Cummings
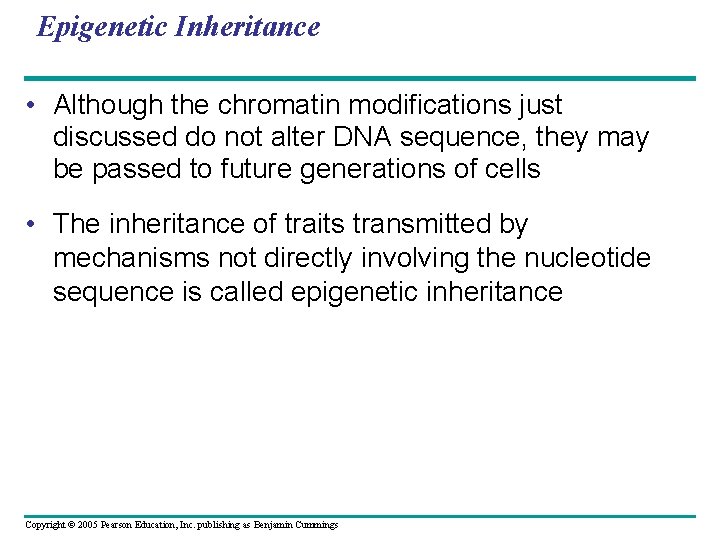
Epigenetic Inheritance • Although the chromatin modifications just discussed do not alter DNA sequence, they may be passed to future generations of cells • The inheritance of traits transmitted by mechanisms not directly involving the nucleotide sequence is called epigenetic inheritance Copyright © 2005 Pearson Education, Inc. publishing as Benjamin Cummings
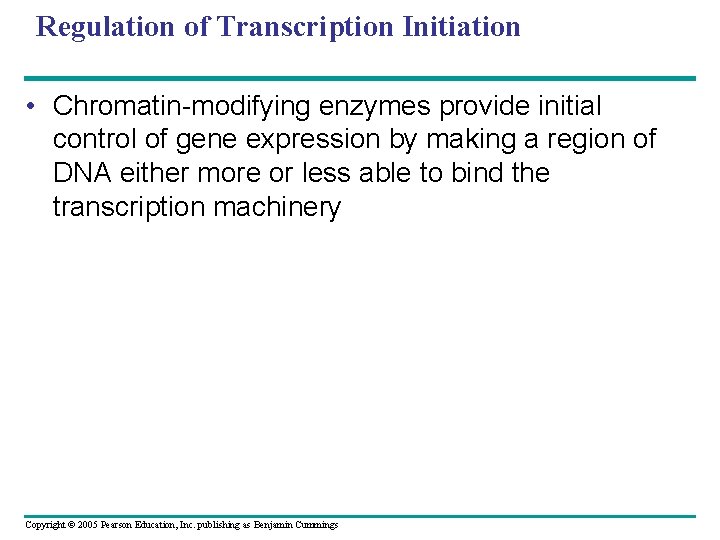
Regulation of Transcription Initiation • Chromatin-modifying enzymes provide initial control of gene expression by making a region of DNA either more or less able to bind the transcription machinery Copyright © 2005 Pearson Education, Inc. publishing as Benjamin Cummings
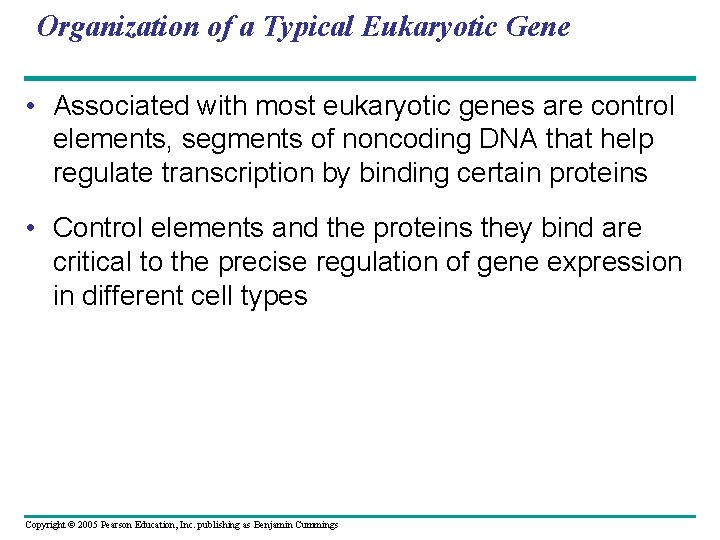
Organization of a Typical Eukaryotic Gene • Associated with most eukaryotic genes are control elements, segments of noncoding DNA that help regulate transcription by binding certain proteins • Control elements and the proteins they bind are critical to the precise regulation of gene expression in different cell types Copyright © 2005 Pearson Education, Inc. publishing as Benjamin Cummings
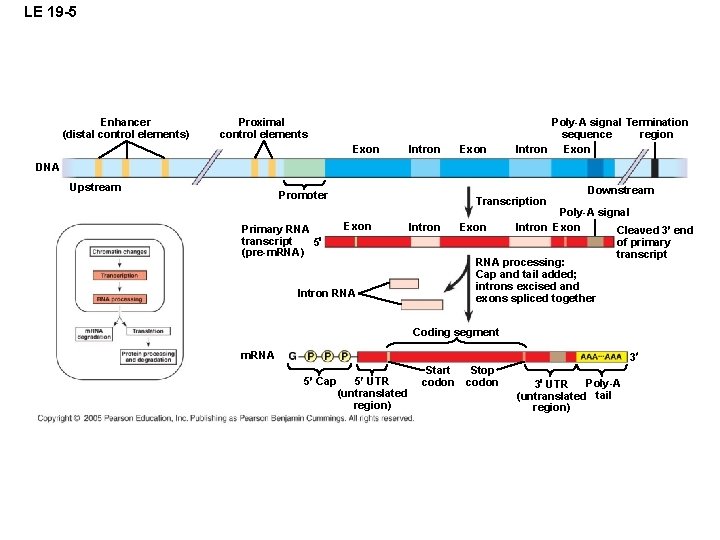
LE 19 -5 Enhancer (distal control elements) Proximal control elements Exon Intron Exon Poly-A signal Termination sequence region Intron Exon DNA Upstream Promoter Primary RNA transcript 5¢ (pre-m. RNA) Transcription Exon Intron RNA Downstream Poly-A signal Exon Intron Exon Cleaved 3¢ end of primary transcript RNA processing: Cap and tail added; introns excised and exons spliced together Coding segment m. RNA 3¢ 5¢ Cap 5¢ UTR (untranslated region) Start codon Stop codon Poly-A 3¢ UTR (untranslated tail region)
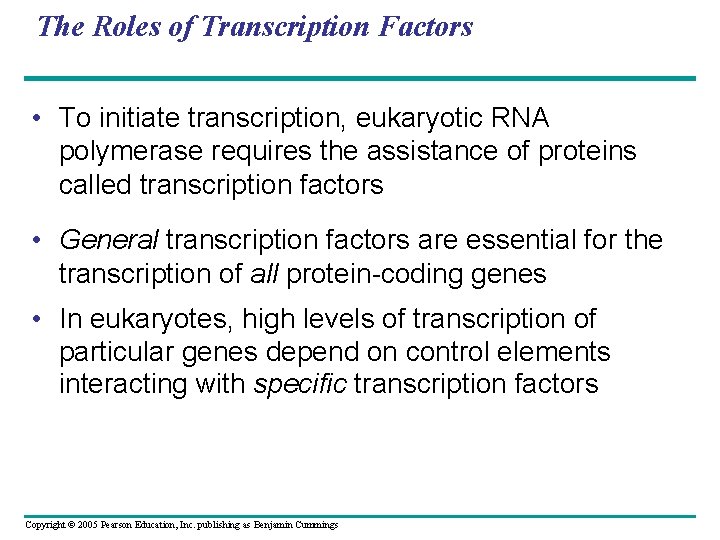
The Roles of Transcription Factors • To initiate transcription, eukaryotic RNA polymerase requires the assistance of proteins called transcription factors • General transcription factors are essential for the transcription of all protein-coding genes • In eukaryotes, high levels of transcription of particular genes depend on control elements interacting with specific transcription factors Copyright © 2005 Pearson Education, Inc. publishing as Benjamin Cummings
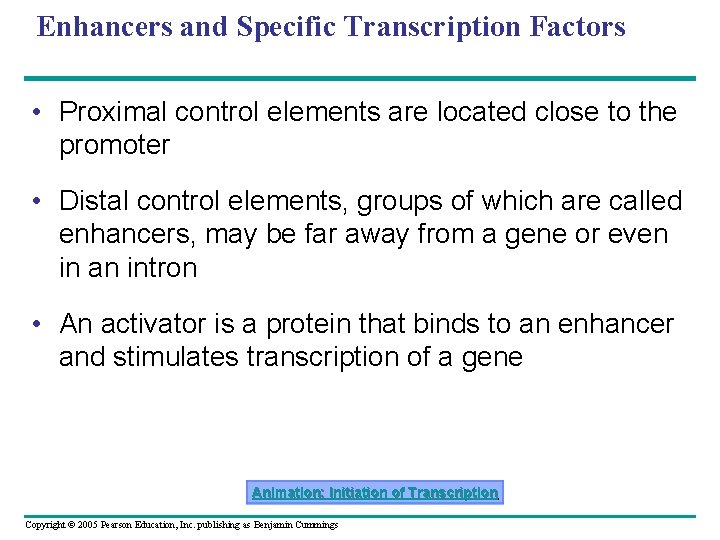
Enhancers and Specific Transcription Factors • Proximal control elements are located close to the promoter • Distal control elements, groups of which are called enhancers, may be far away from a gene or even in an intron • An activator is a protein that binds to an enhancer and stimulates transcription of a gene Animation: Initiation of Transcription Copyright © 2005 Pearson Education, Inc. publishing as Benjamin Cummings
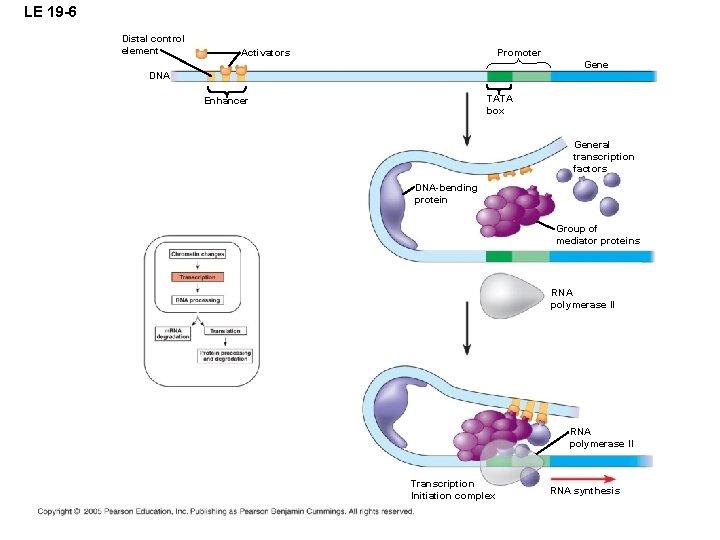
LE 19 -6 Distal control element Activators Promoter Gene DNA TATA box Enhancer General transcription factors DNA-bending protein Group of mediator proteins RNA polymerase II Transcription Initiation complex RNA synthesis
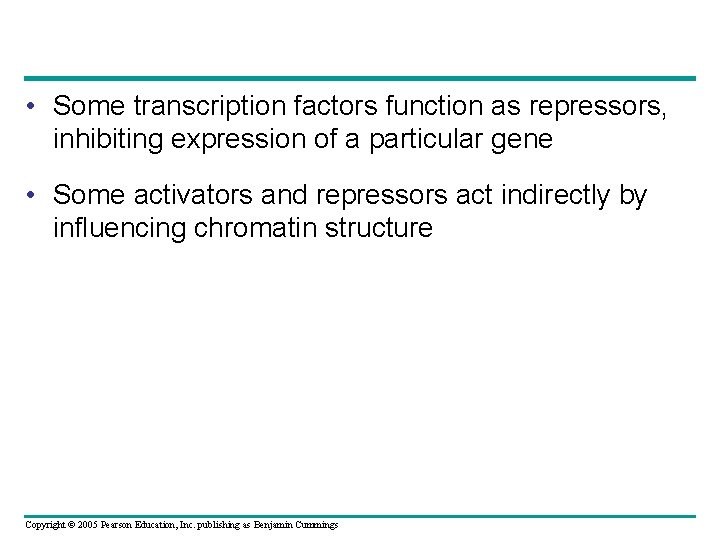
• Some transcription factors function as repressors, inhibiting expression of a particular gene • Some activators and repressors act indirectly by influencing chromatin structure Copyright © 2005 Pearson Education, Inc. publishing as Benjamin Cummings
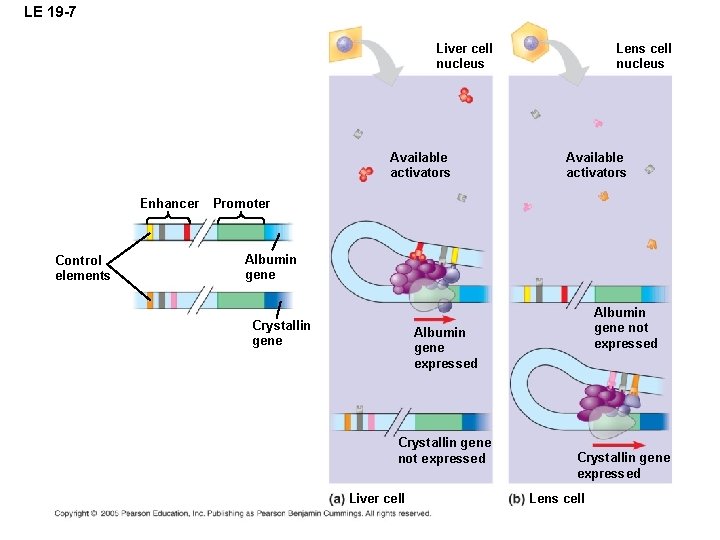
LE 19 -7 Liver cell nucleus Available activators Enhancer Control elements Lens cell nucleus Available activators Promoter Albumin gene Crystallin gene Albumin gene not expressed Albumin gene expressed Crystallin gene not expressed Liver cell Crystallin gene expressed Lens cell
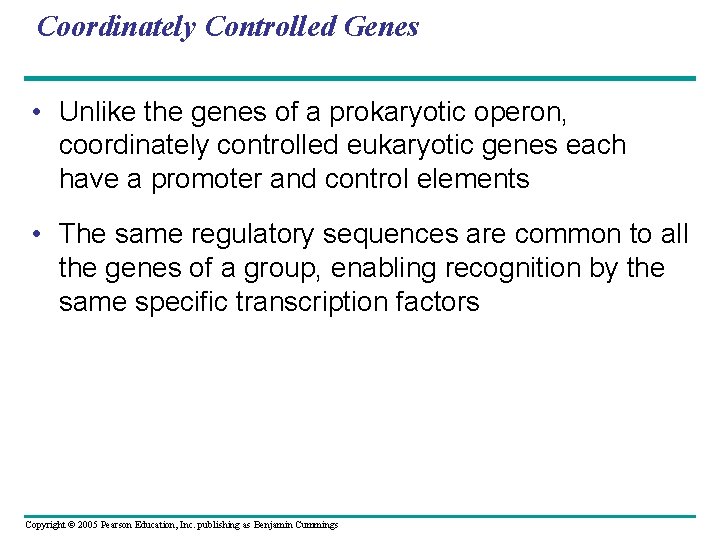
Coordinately Controlled Genes • Unlike the genes of a prokaryotic operon, coordinately controlled eukaryotic genes each have a promoter and control elements • The same regulatory sequences are common to all the genes of a group, enabling recognition by the same specific transcription factors Copyright © 2005 Pearson Education, Inc. publishing as Benjamin Cummings
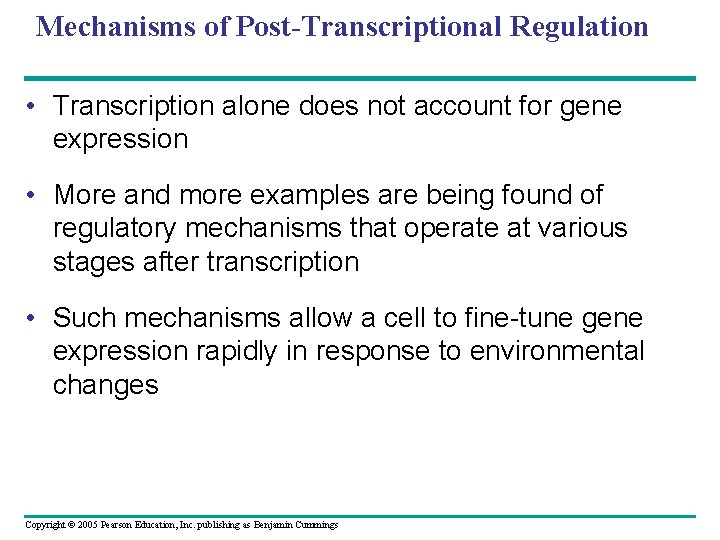
Mechanisms of Post-Transcriptional Regulation • Transcription alone does not account for gene expression • More and more examples are being found of regulatory mechanisms that operate at various stages after transcription • Such mechanisms allow a cell to fine-tune gene expression rapidly in response to environmental changes Copyright © 2005 Pearson Education, Inc. publishing as Benjamin Cummings
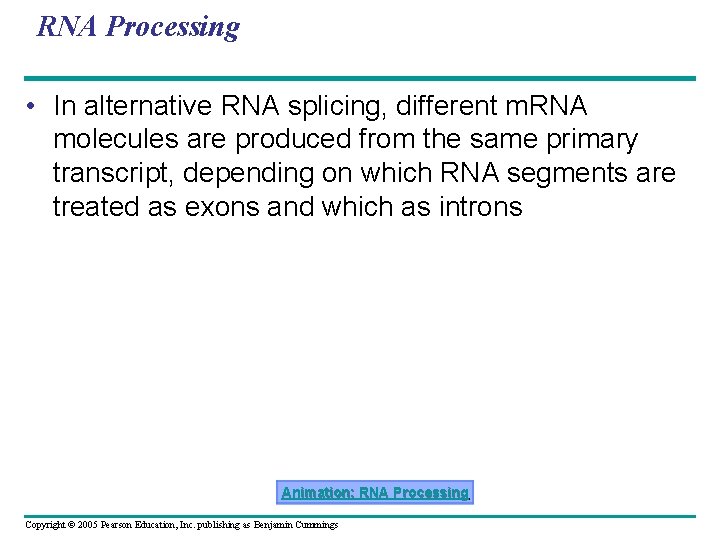
RNA Processing • In alternative RNA splicing, different m. RNA molecules are produced from the same primary transcript, depending on which RNA segments are treated as exons and which as introns Animation: RNA Processing Copyright © 2005 Pearson Education, Inc. publishing as Benjamin Cummings
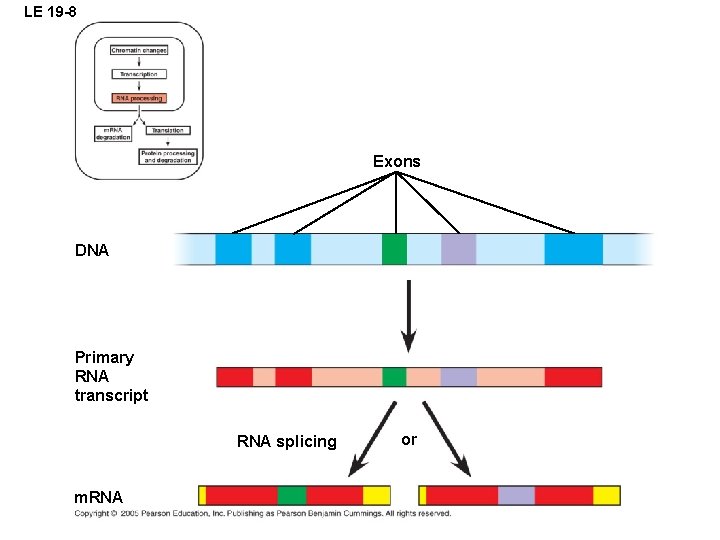
LE 19 -8 Exons DNA Primary RNA transcript RNA splicing m. RNA or
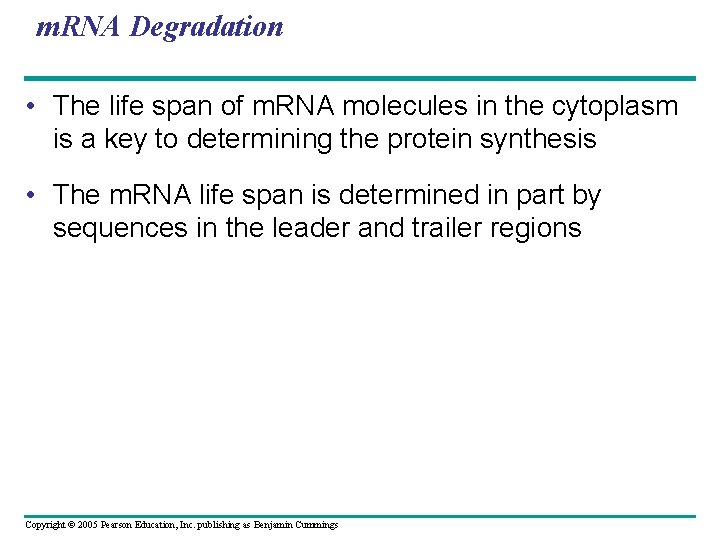
m. RNA Degradation • The life span of m. RNA molecules in the cytoplasm is a key to determining the protein synthesis • The m. RNA life span is determined in part by sequences in the leader and trailer regions Copyright © 2005 Pearson Education, Inc. publishing as Benjamin Cummings
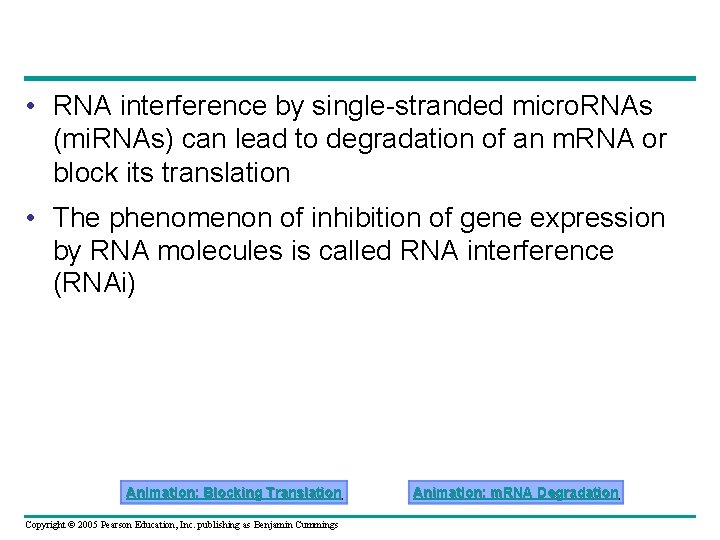
• RNA interference by single-stranded micro. RNAs (mi. RNAs) can lead to degradation of an m. RNA or block its translation • The phenomenon of inhibition of gene expression by RNA molecules is called RNA interference (RNAi) Animation: Blocking Translation Copyright © 2005 Pearson Education, Inc. publishing as Benjamin Cummings Animation: m. RNA Degradation
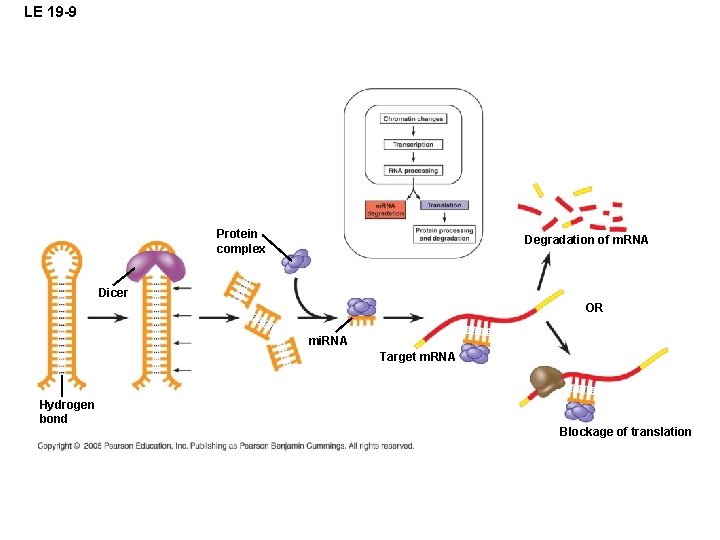
LE 19 -9 Protein complex Degradation of m. RNA Dicer OR mi. RNA Target m. RNA Hydrogen bond Blockage of translation
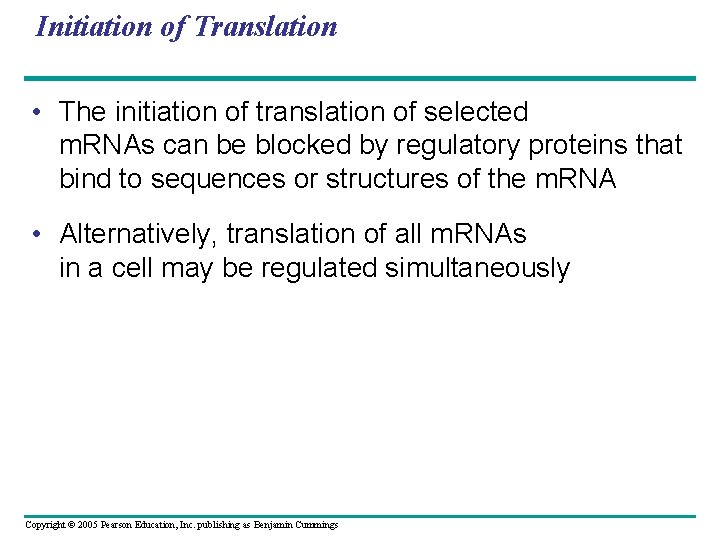
Initiation of Translation • The initiation of translation of selected m. RNAs can be blocked by regulatory proteins that bind to sequences or structures of the m. RNA • Alternatively, translation of all m. RNAs in a cell may be regulated simultaneously Copyright © 2005 Pearson Education, Inc. publishing as Benjamin Cummings
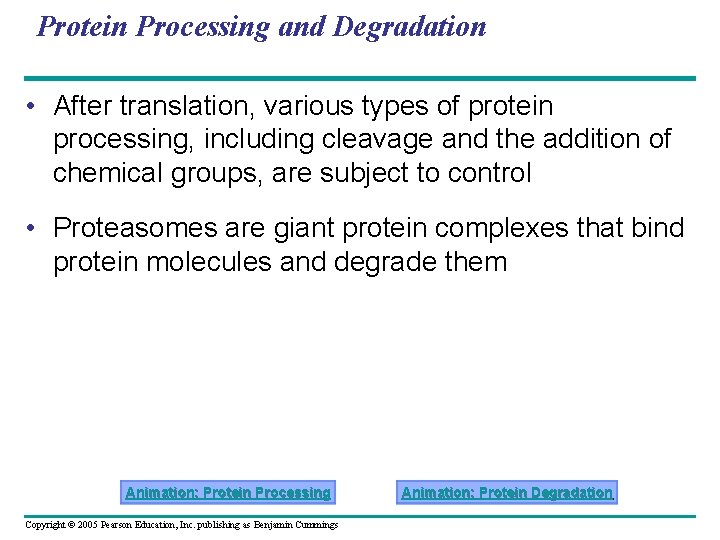
Protein Processing and Degradation • After translation, various types of protein processing, including cleavage and the addition of chemical groups, are subject to control • Proteasomes are giant protein complexes that bind protein molecules and degrade them Animation: Protein Processing Copyright © 2005 Pearson Education, Inc. publishing as Benjamin Cummings Animation: Protein Degradation
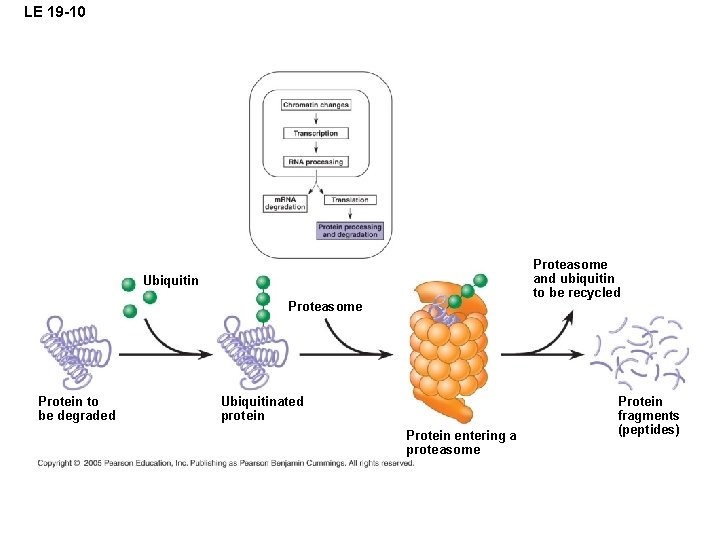
LE 19 -10 Proteasome and ubiquitin to be recycled Ubiquitin Proteasome Protein to be degraded Ubiquitinated protein Protein entering a proteasome Protein fragments (peptides)
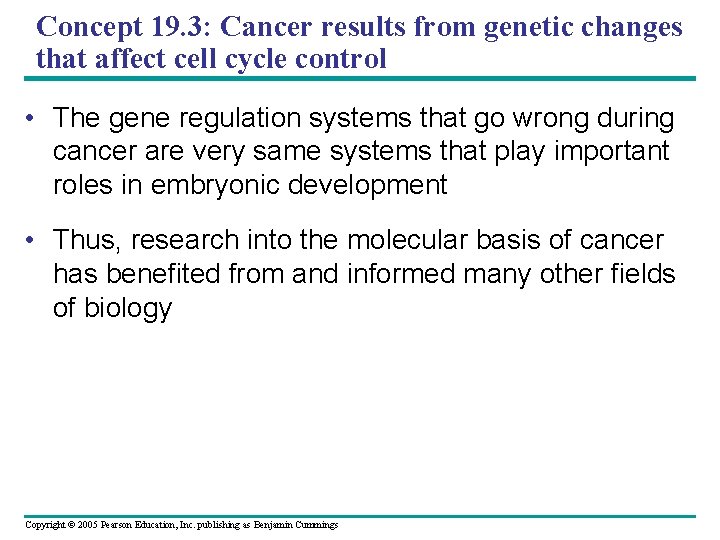
Concept 19. 3: Cancer results from genetic changes that affect cell cycle control • The gene regulation systems that go wrong during cancer are very same systems that play important roles in embryonic development • Thus, research into the molecular basis of cancer has benefited from and informed many other fields of biology Copyright © 2005 Pearson Education, Inc. publishing as Benjamin Cummings
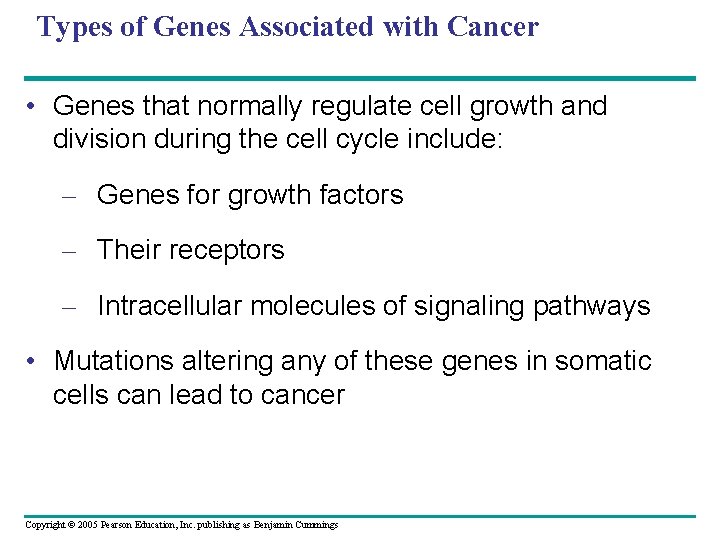
Types of Genes Associated with Cancer • Genes that normally regulate cell growth and division during the cell cycle include: – Genes for growth factors – Their receptors – Intracellular molecules of signaling pathways • Mutations altering any of these genes in somatic cells can lead to cancer Copyright © 2005 Pearson Education, Inc. publishing as Benjamin Cummings
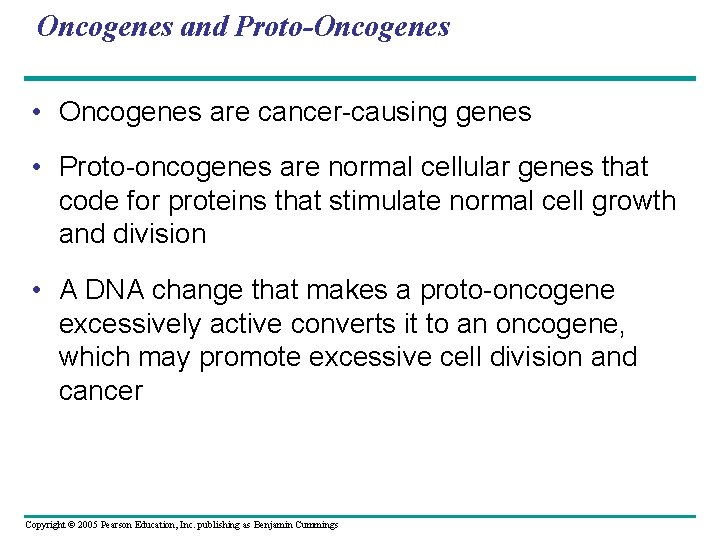
Oncogenes and Proto-Oncogenes • Oncogenes are cancer-causing genes • Proto-oncogenes are normal cellular genes that code for proteins that stimulate normal cell growth and division • A DNA change that makes a proto-oncogene excessively active converts it to an oncogene, which may promote excessive cell division and cancer Copyright © 2005 Pearson Education, Inc. publishing as Benjamin Cummings
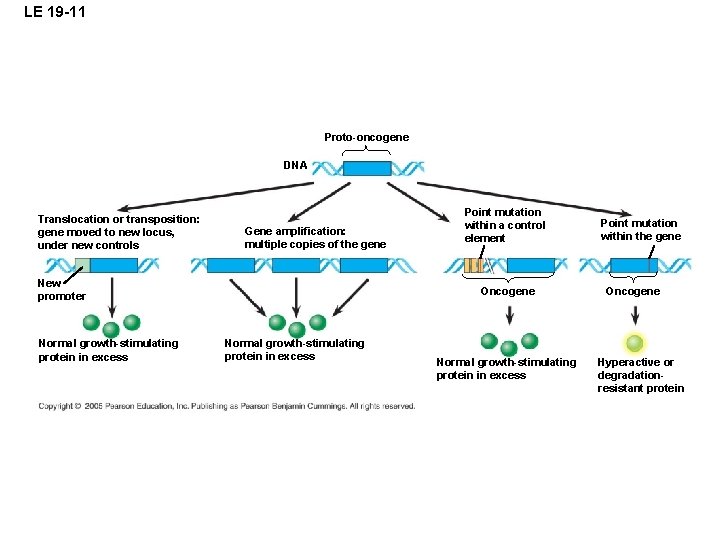
LE 19 -11 Proto-oncogene DNA Translocation or transposition: gene moved to new locus, under new controls Gene amplification: multiple copies of the gene New promoter Normal growth-stimulating protein in excess Point mutation within a control element Oncogene Normal growth-stimulating protein in excess Point mutation within the gene Oncogene Hyperactive or degradationresistant protein
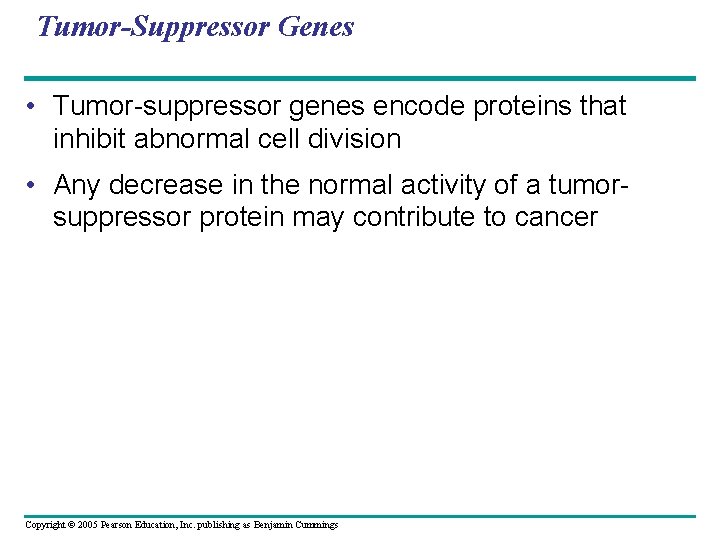
Tumor-Suppressor Genes • Tumor-suppressor genes encode proteins that inhibit abnormal cell division • Any decrease in the normal activity of a tumorsuppressor protein may contribute to cancer Copyright © 2005 Pearson Education, Inc. publishing as Benjamin Cummings
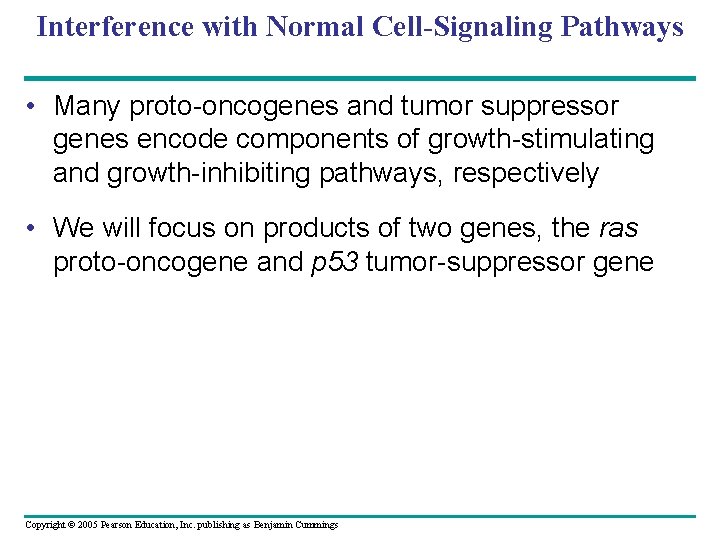
Interference with Normal Cell-Signaling Pathways • Many proto-oncogenes and tumor suppressor genes encode components of growth-stimulating and growth-inhibiting pathways, respectively • We will focus on products of two genes, the ras proto-oncogene and p 53 tumor-suppressor gene Copyright © 2005 Pearson Education, Inc. publishing as Benjamin Cummings
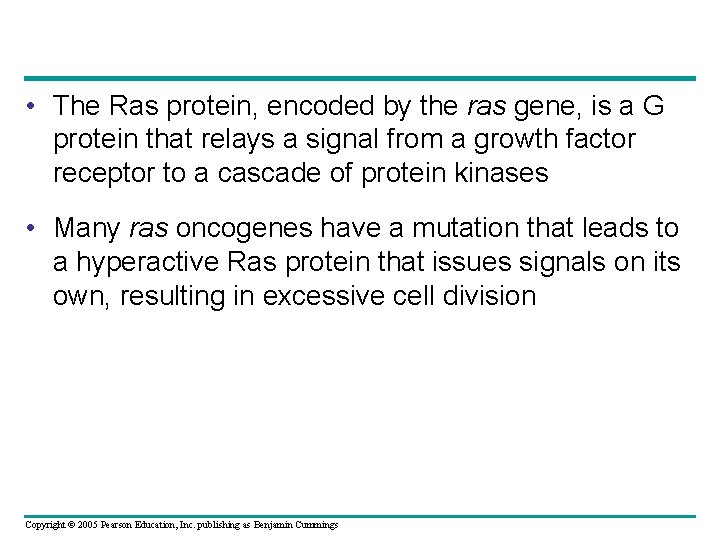
• The Ras protein, encoded by the ras gene, is a G protein that relays a signal from a growth factor receptor to a cascade of protein kinases • Many ras oncogenes have a mutation that leads to a hyperactive Ras protein that issues signals on its own, resulting in excessive cell division Copyright © 2005 Pearson Education, Inc. publishing as Benjamin Cummings
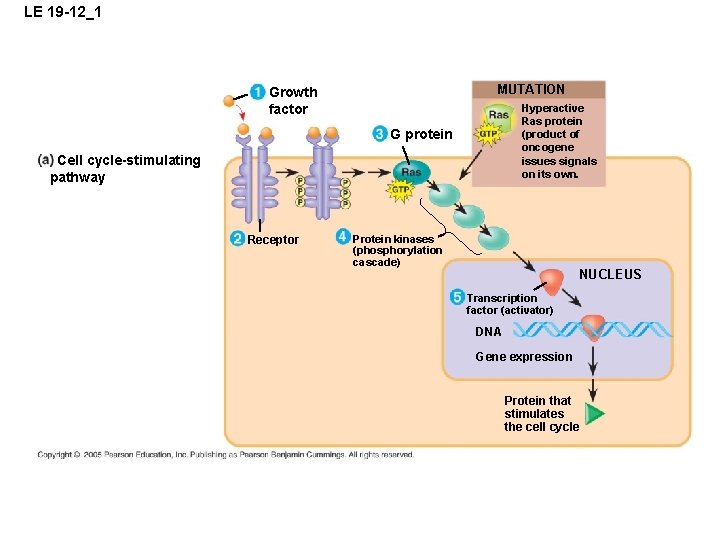
LE 19 -12_1 MUTATION Growth factor Hyperactive Ras protein (product of oncogene issues signals on its own. G protein Cell cycle-stimulating pathway Receptor Protein kinases (phosphorylation cascade) NUCLEUS Transcription factor (activator) DNA Gene expression Protein that stimulates the cell cycle
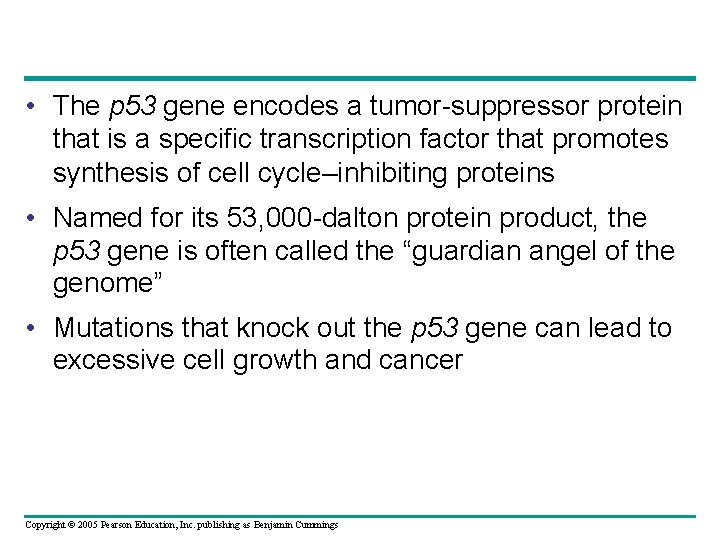
• The p 53 gene encodes a tumor-suppressor protein that is a specific transcription factor that promotes synthesis of cell cycle–inhibiting proteins • Named for its 53, 000 -dalton protein product, the p 53 gene is often called the “guardian angel of the genome” • Mutations that knock out the p 53 gene can lead to excessive cell growth and cancer Copyright © 2005 Pearson Education, Inc. publishing as Benjamin Cummings
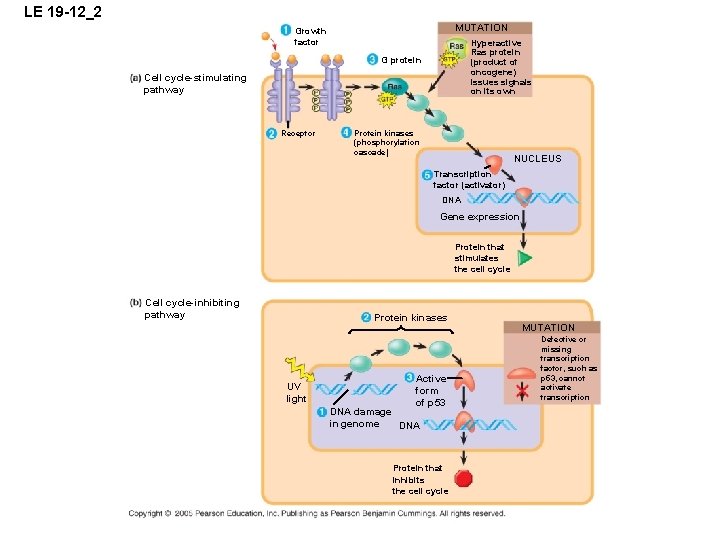
LE 19 -12_2 MUTATION Growth factor Hyperactive Ras protein (product of oncogene) issues signals on its own G protein Cell cycle-stimulating pathway Receptor Protein kinases (phosphorylation cascade) NUCLEUS Transcription factor (activator) DNA Gene expression Protein that stimulates the cell cycle Cell cycle-inhibiting pathway Protein kinases UV light Active form of p 53 DNA damage in genome DNA Protein that inhibits the cell cycle MUTATION Defective or missing transcription factor, such as p 53, cannot activate transcription
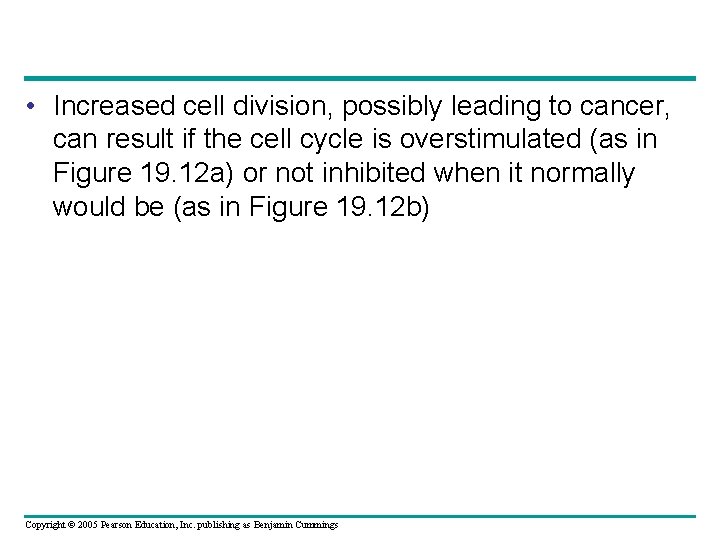
• Increased cell division, possibly leading to cancer, can result if the cell cycle is overstimulated (as in Figure 19. 12 a) or not inhibited when it normally would be (as in Figure 19. 12 b) Copyright © 2005 Pearson Education, Inc. publishing as Benjamin Cummings
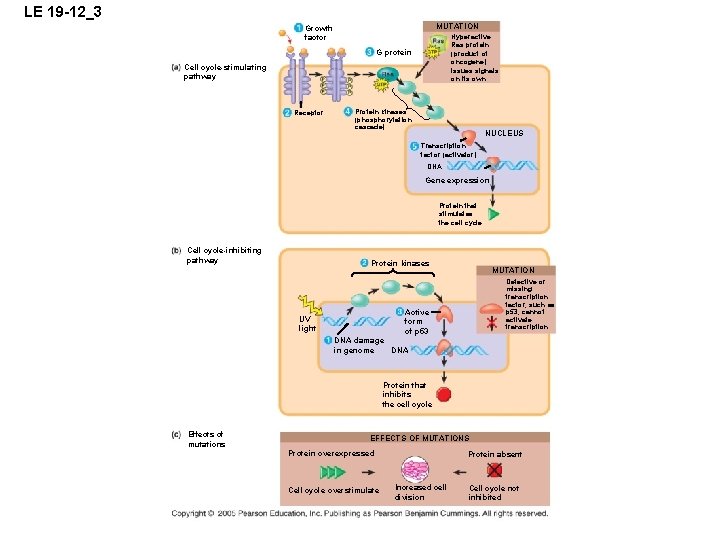
LE 19 -12_3 MUTATION Growth factor Hyperactive Ras protein (product of oncogene) issues signals on its own G protein Cell cycle-stimulating pathway Receptor Protein kinases (phosphorylation cascade) NUCLEUS Transcription factor (activator) DNA Gene expression Protein that stimulates the cell cycle Cell cycle-inhibiting pathway Protein kinases MUTATION Defective or missing transcription factor, such as p 53, cannot activate transcription Active form of p 53 UV light DNA damage in genome DNA Protein that inhibits the cell cycle Effects of mutations EFFECTS OF MUTATIONS Protein overexpressed Cell cycle overstimulate Protein absent Increased cell division Cell cycle not inhibited
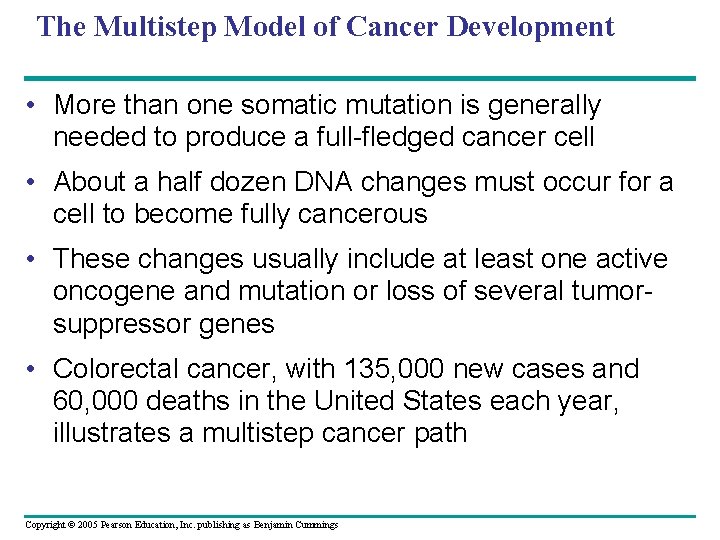
The Multistep Model of Cancer Development • More than one somatic mutation is generally needed to produce a full-fledged cancer cell • About a half dozen DNA changes must occur for a cell to become fully cancerous • These changes usually include at least one active oncogene and mutation or loss of several tumorsuppressor genes • Colorectal cancer, with 135, 000 new cases and 60, 000 deaths in the United States each year, illustrates a multistep cancer path Copyright © 2005 Pearson Education, Inc. publishing as Benjamin Cummings
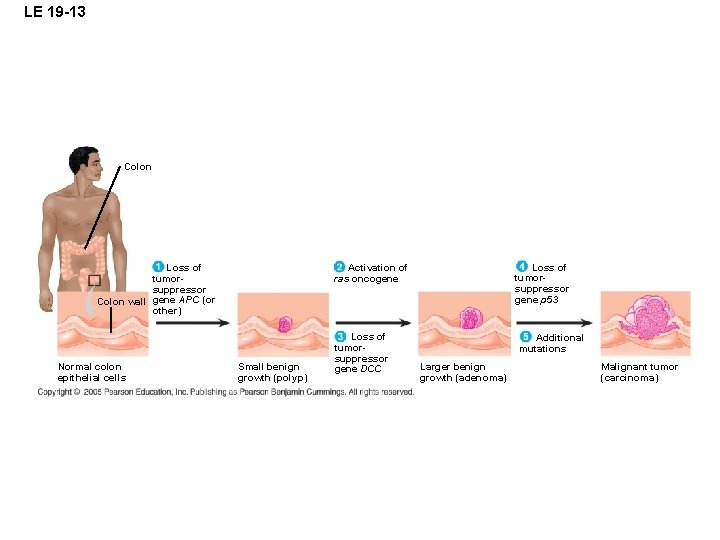
LE 19 -13 Colon Normal colon epithelial cells Loss of tumorsuppressor gene p 53 Activation of ras oncogene Loss of tumorsuppressor Colon wall gene APC (or other) Small benign growth (polyp) Loss of tumorsuppressor gene DCC Additional mutations Larger benign growth (adenoma) Malignant tumor (carcinoma)
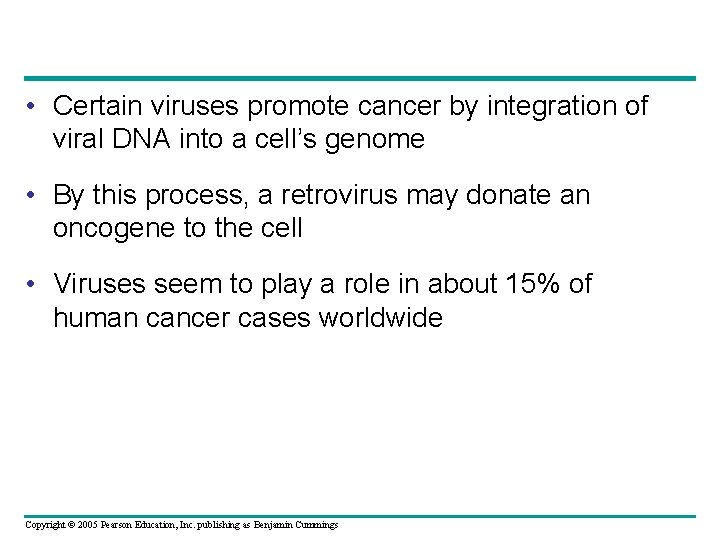
• Certain viruses promote cancer by integration of viral DNA into a cell’s genome • By this process, a retrovirus may donate an oncogene to the cell • Viruses seem to play a role in about 15% of human cancer cases worldwide Copyright © 2005 Pearson Education, Inc. publishing as Benjamin Cummings
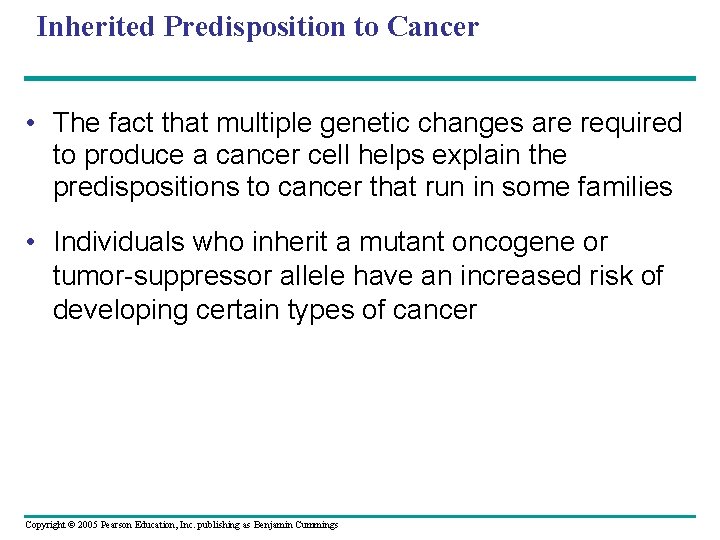
Inherited Predisposition to Cancer • The fact that multiple genetic changes are required to produce a cancer cell helps explain the predispositions to cancer that run in some families • Individuals who inherit a mutant oncogene or tumor-suppressor allele have an increased risk of developing certain types of cancer Copyright © 2005 Pearson Education, Inc. publishing as Benjamin Cummings
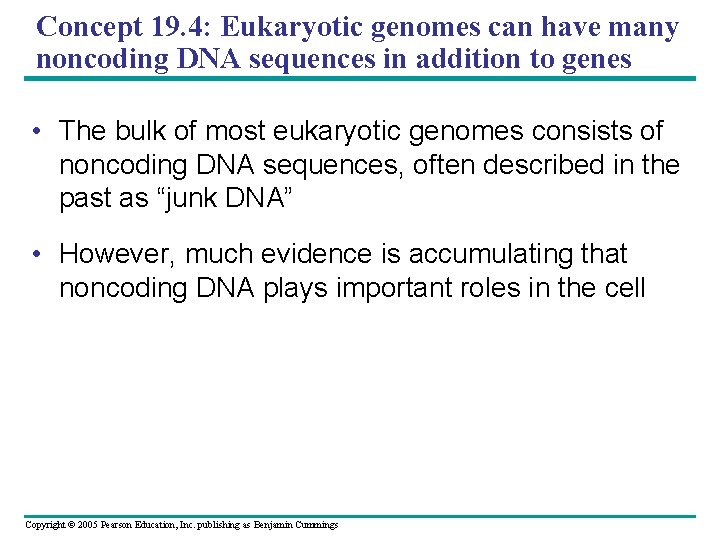
Concept 19. 4: Eukaryotic genomes can have many noncoding DNA sequences in addition to genes • The bulk of most eukaryotic genomes consists of noncoding DNA sequences, often described in the past as “junk DNA” • However, much evidence is accumulating that noncoding DNA plays important roles in the cell Copyright © 2005 Pearson Education, Inc. publishing as Benjamin Cummings
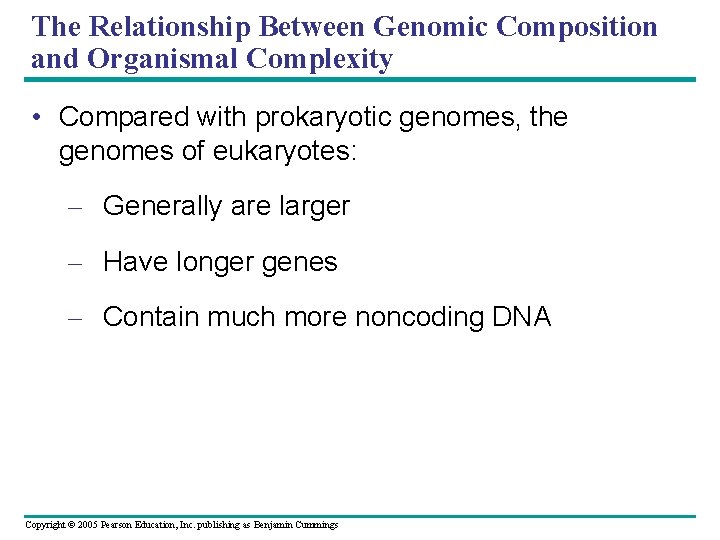
The Relationship Between Genomic Composition and Organismal Complexity • Compared with prokaryotic genomes, the genomes of eukaryotes: – Generally are larger – Have longer genes – Contain much more noncoding DNA Copyright © 2005 Pearson Education, Inc. publishing as Benjamin Cummings
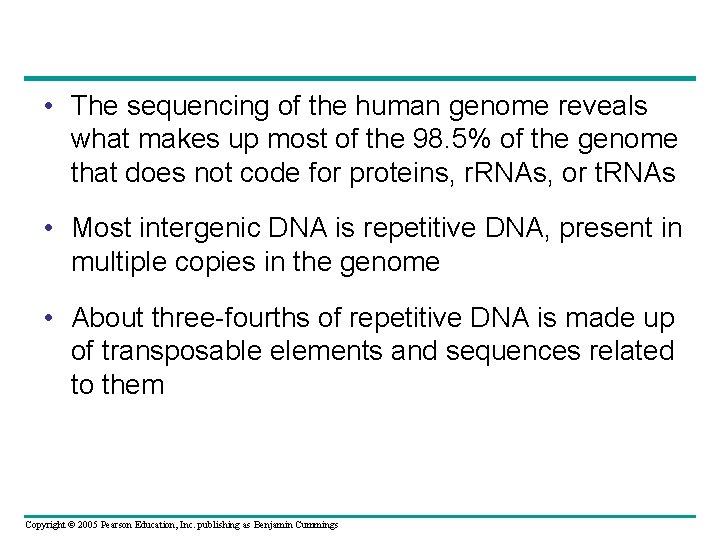
• The sequencing of the human genome reveals what makes up most of the 98. 5% of the genome that does not code for proteins, r. RNAs, or t. RNAs • Most intergenic DNA is repetitive DNA, present in multiple copies in the genome • About three-fourths of repetitive DNA is made up of transposable elements and sequences related to them Copyright © 2005 Pearson Education, Inc. publishing as Benjamin Cummings
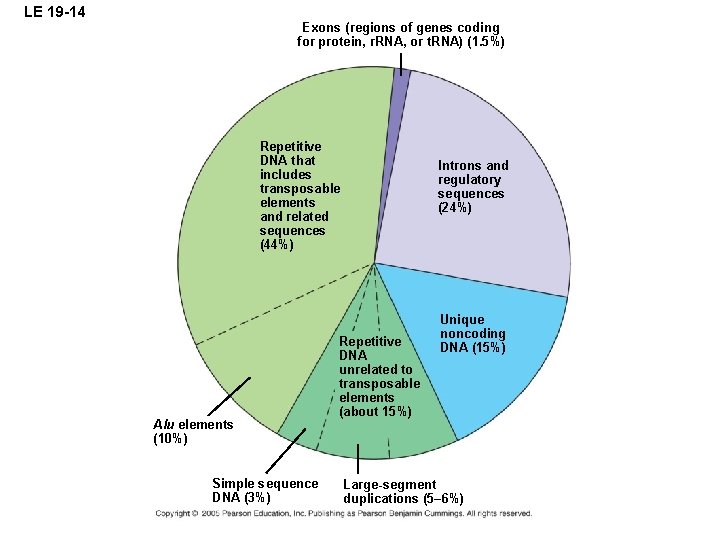
LE 19 -14 Exons (regions of genes coding for protein, r. RNA, or t. RNA) (1. 5%) Repetitive DNA that includes transposable elements and related sequences (44%) Alu elements (10%) Simple sequence DNA (3%) Introns and regulatory sequences (24%) Repetitive DNA unrelated to transposable elements (about 15%) Unique noncoding DNA (15%) Large-segment duplications (5– 6%)
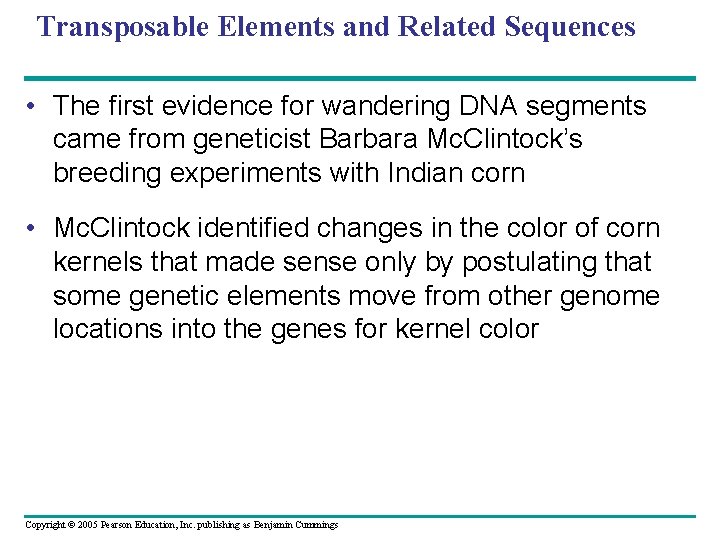
Transposable Elements and Related Sequences • The first evidence for wandering DNA segments came from geneticist Barbara Mc. Clintock’s breeding experiments with Indian corn • Mc. Clintock identified changes in the color of corn kernels that made sense only by postulating that some genetic elements move from other genome locations into the genes for kernel color Copyright © 2005 Pearson Education, Inc. publishing as Benjamin Cummings
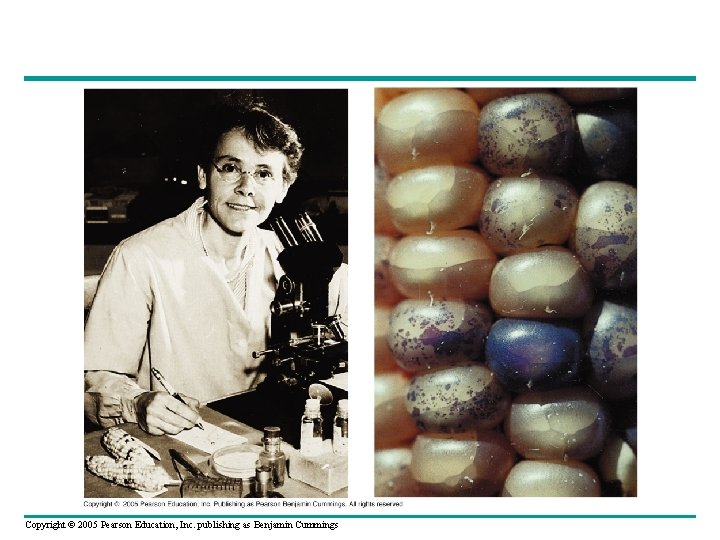
Copyright © 2005 Pearson Education, Inc. publishing as Benjamin Cummings
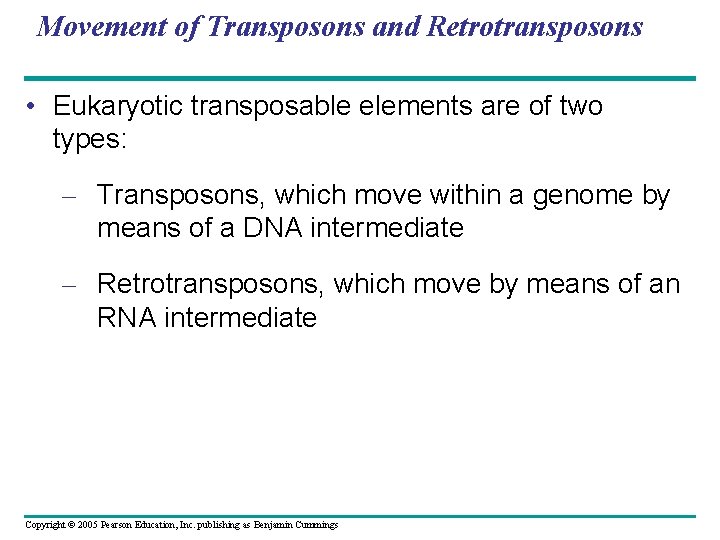
Movement of Transposons and Retrotransposons • Eukaryotic transposable elements are of two types: – Transposons, which move within a genome by means of a DNA intermediate – Retrotransposons, which move by means of an RNA intermediate Copyright © 2005 Pearson Education, Inc. publishing as Benjamin Cummings
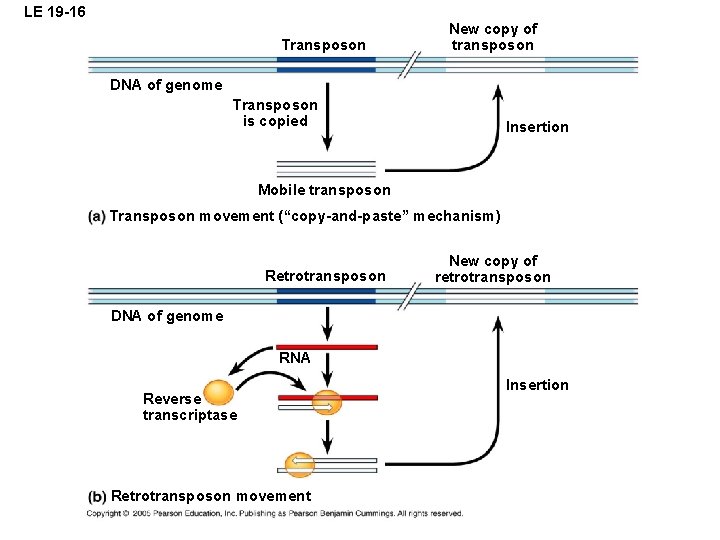
LE 19 -16 Transposon New copy of transposon DNA of genome Transposon is copied Insertion Mobile transposon Transposon movement (“copy-and-paste” mechanism) Retrotransposon New copy of retrotransposon DNA of genome RNA Reverse transcriptase Retrotransposon movement Insertion
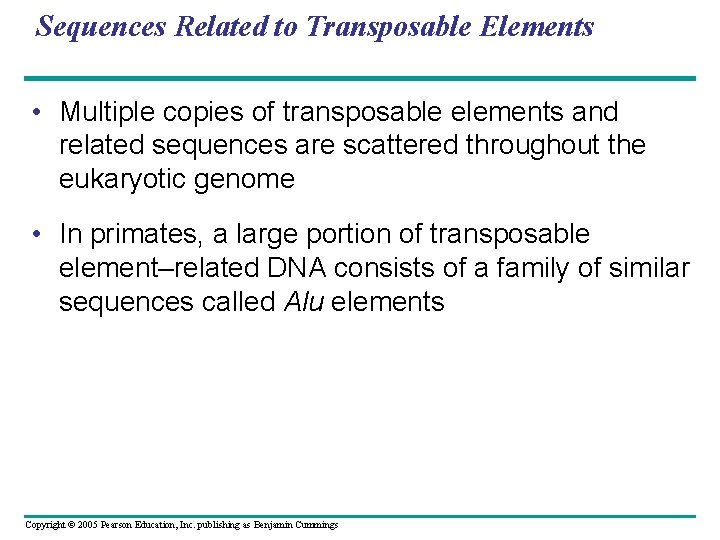
Sequences Related to Transposable Elements • Multiple copies of transposable elements and related sequences are scattered throughout the eukaryotic genome • In primates, a large portion of transposable element–related DNA consists of a family of similar sequences called Alu elements Copyright © 2005 Pearson Education, Inc. publishing as Benjamin Cummings
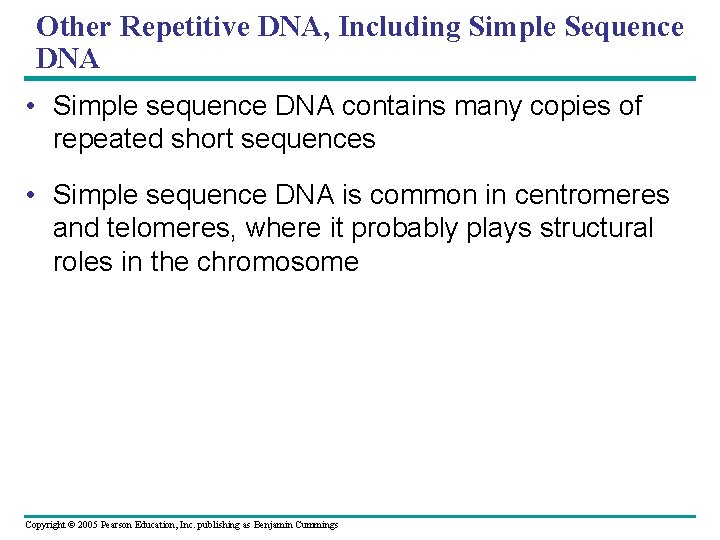
Other Repetitive DNA, Including Simple Sequence DNA • Simple sequence DNA contains many copies of repeated short sequences • Simple sequence DNA is common in centromeres and telomeres, where it probably plays structural roles in the chromosome Copyright © 2005 Pearson Education, Inc. publishing as Benjamin Cummings
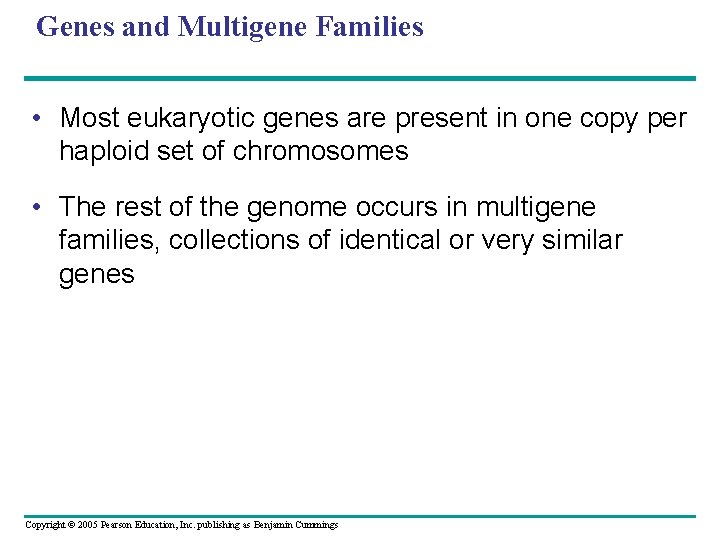
Genes and Multigene Families • Most eukaryotic genes are present in one copy per haploid set of chromosomes • The rest of the genome occurs in multigene families, collections of identical or very similar genes Copyright © 2005 Pearson Education, Inc. publishing as Benjamin Cummings
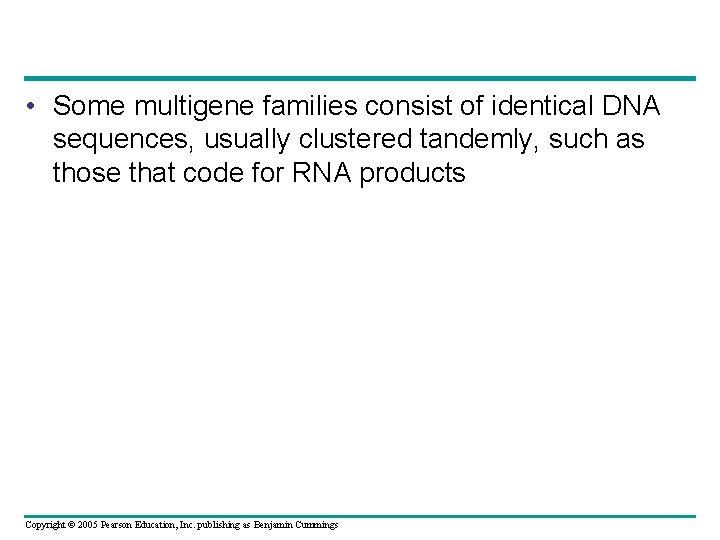
• Some multigene families consist of identical DNA sequences, usually clustered tandemly, such as those that code for RNA products Copyright © 2005 Pearson Education, Inc. publishing as Benjamin Cummings
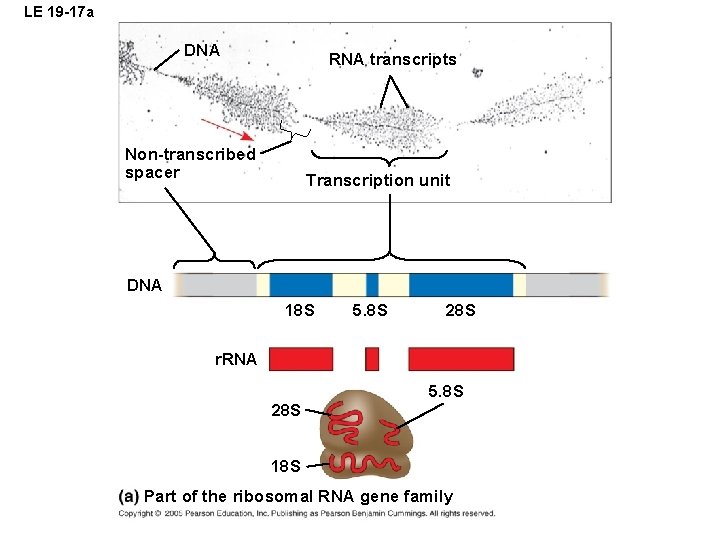
LE 19 -17 a DNA RNA transcripts Non-transcribed spacer Transcription unit DNA 18 S 5. 8 S 28 S r. RNA 28 S 5. 8 S 18 S Part of the ribosomal RNA gene family
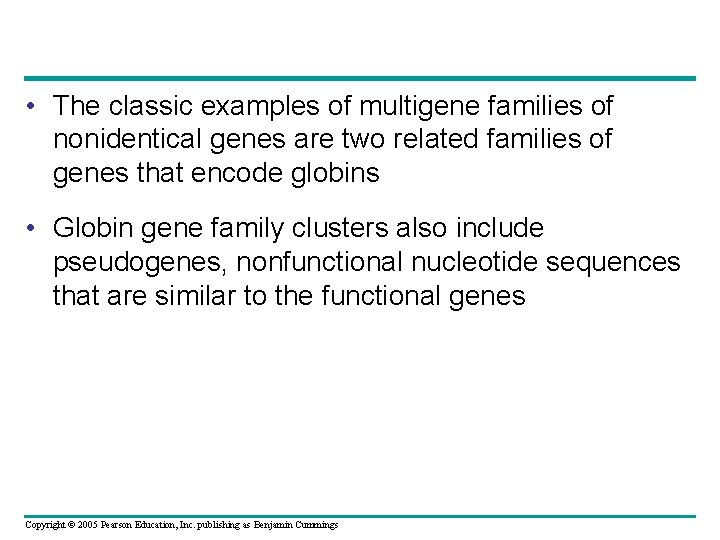
• The classic examples of multigene families of nonidentical genes are two related families of genes that encode globins • Globin gene family clusters also include pseudogenes, nonfunctional nucleotide sequences that are similar to the functional genes Copyright © 2005 Pearson Education, Inc. publishing as Benjamin Cummings
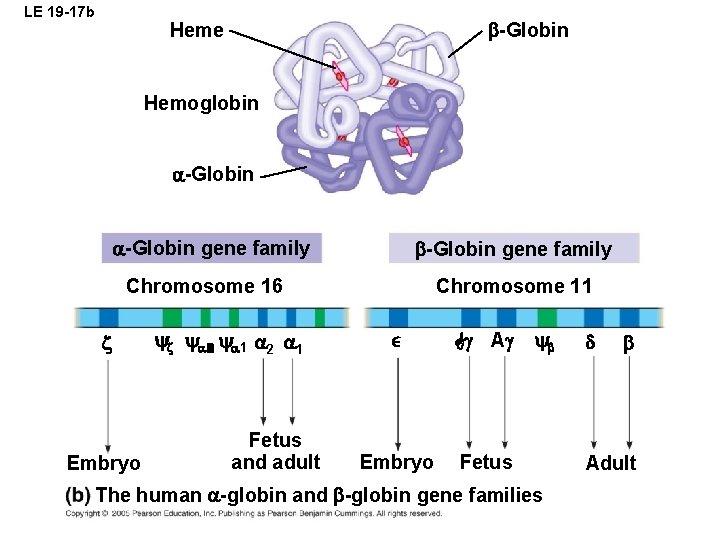
LE 19 -17 b -Globin Heme Hemoglobin -Globin gene family Chromosome 11 Chromosome 16 Embryo 1 2 1 Fetus and adult A Embryo Fetus The human -globin and -globin gene families Adult
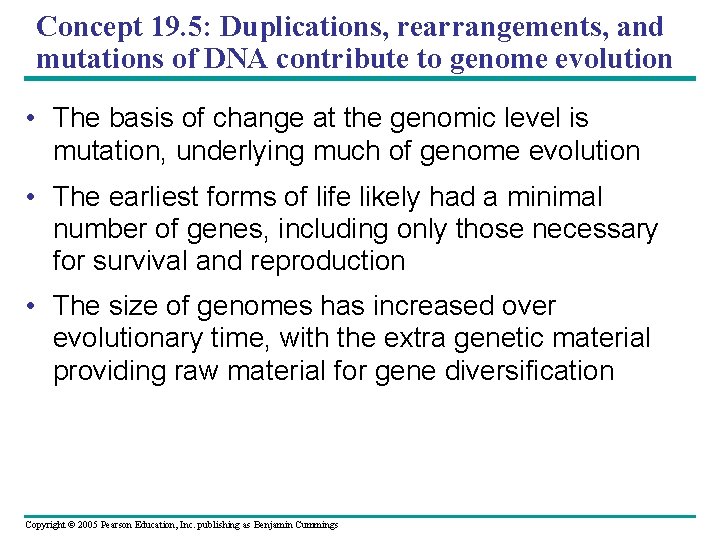
Concept 19. 5: Duplications, rearrangements, and mutations of DNA contribute to genome evolution • The basis of change at the genomic level is mutation, underlying much of genome evolution • The earliest forms of life likely had a minimal number of genes, including only those necessary for survival and reproduction • The size of genomes has increased over evolutionary time, with the extra genetic material providing raw material for gene diversification Copyright © 2005 Pearson Education, Inc. publishing as Benjamin Cummings
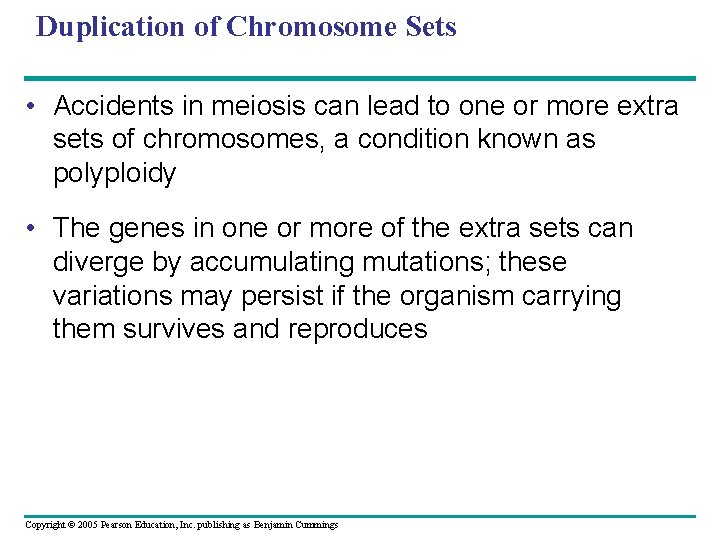
Duplication of Chromosome Sets • Accidents in meiosis can lead to one or more extra sets of chromosomes, a condition known as polyploidy • The genes in one or more of the extra sets can diverge by accumulating mutations; these variations may persist if the organism carrying them survives and reproduces Copyright © 2005 Pearson Education, Inc. publishing as Benjamin Cummings
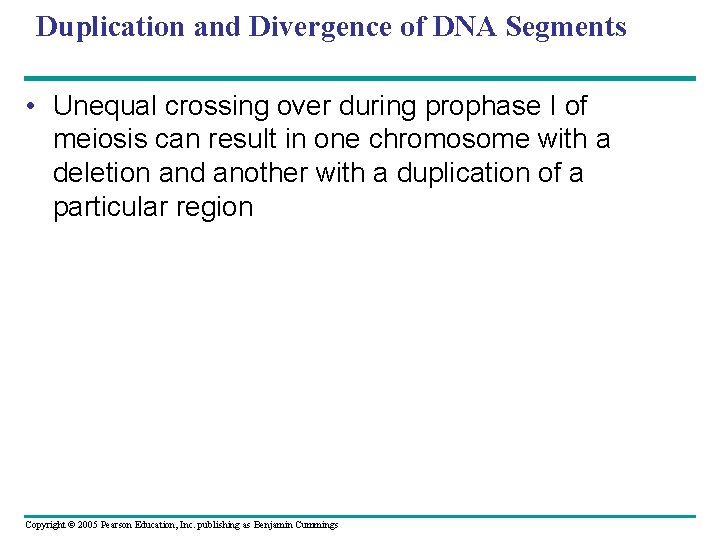
Duplication and Divergence of DNA Segments • Unequal crossing over during prophase I of meiosis can result in one chromosome with a deletion and another with a duplication of a particular region Copyright © 2005 Pearson Education, Inc. publishing as Benjamin Cummings
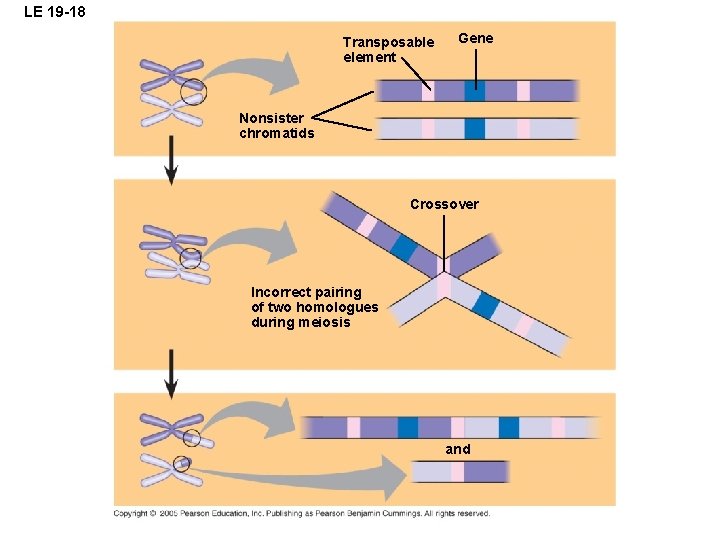
LE 19 -18 Transposable element Gene Nonsister chromatids Crossover Incorrect pairing of two homologues during meiosis and
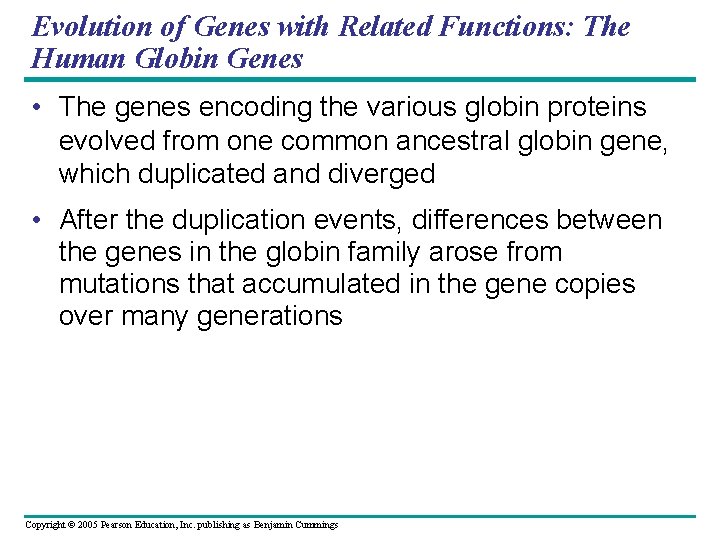
Evolution of Genes with Related Functions: The Human Globin Genes • The genes encoding the various globin proteins evolved from one common ancestral globin gene, which duplicated and diverged • After the duplication events, differences between the genes in the globin family arose from mutations that accumulated in the gene copies over many generations Copyright © 2005 Pearson Education, Inc. publishing as Benjamin Cummings
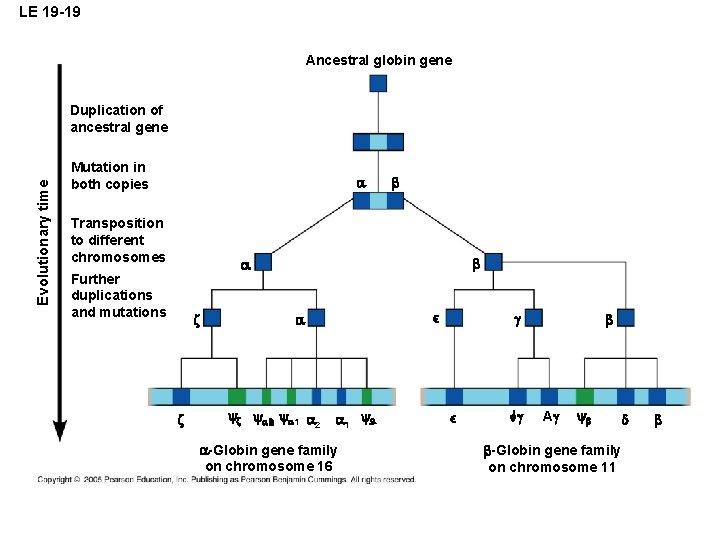
LE 19 -19 Ancestral globin gene Mutation in both copies Transposition to different chromosomes 1 2 1 -Globin gene family on chromosome 16 Further duplications and mutations Evolutionary time Duplication of ancestral gene A -Globin gene family on chromosome 11
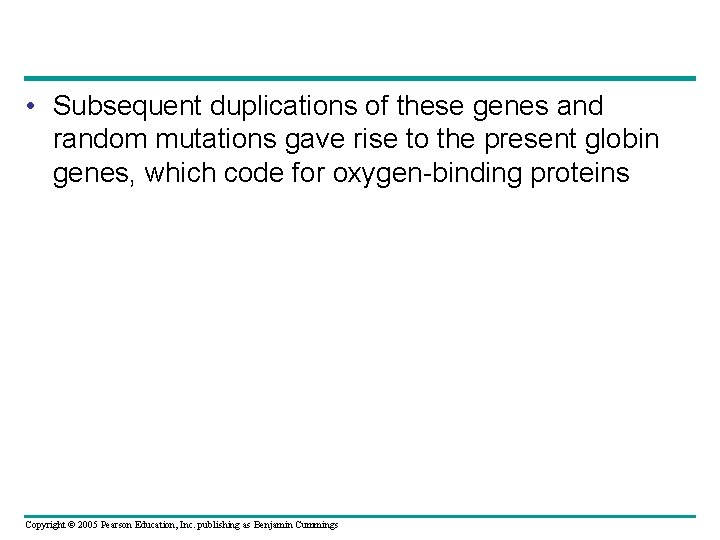
• Subsequent duplications of these genes and random mutations gave rise to the present globin genes, which code for oxygen-binding proteins Copyright © 2005 Pearson Education, Inc. publishing as Benjamin Cummings
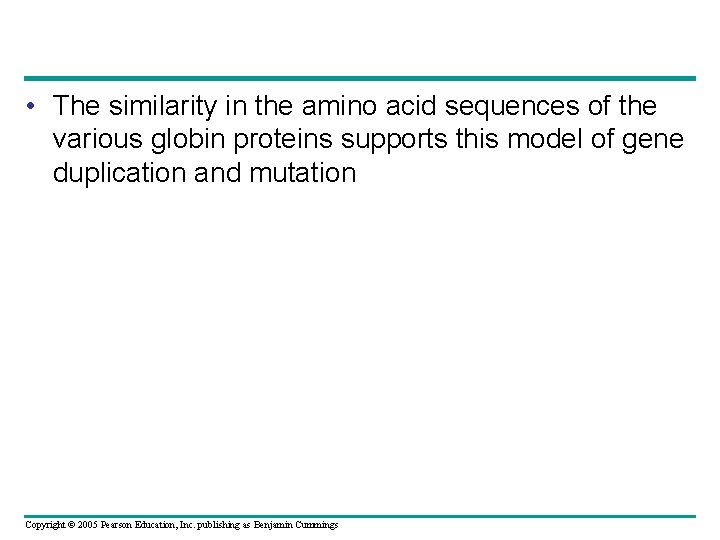
• The similarity in the amino acid sequences of the various globin proteins supports this model of gene duplication and mutation Copyright © 2005 Pearson Education, Inc. publishing as Benjamin Cummings
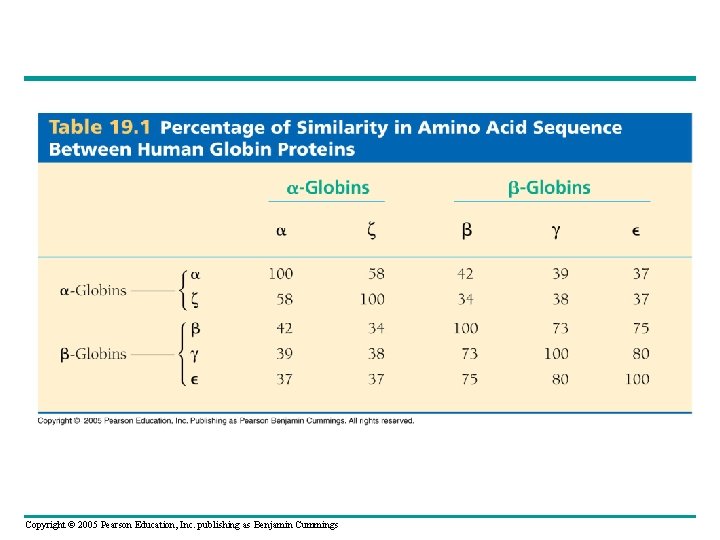
Copyright © 2005 Pearson Education, Inc. publishing as Benjamin Cummings
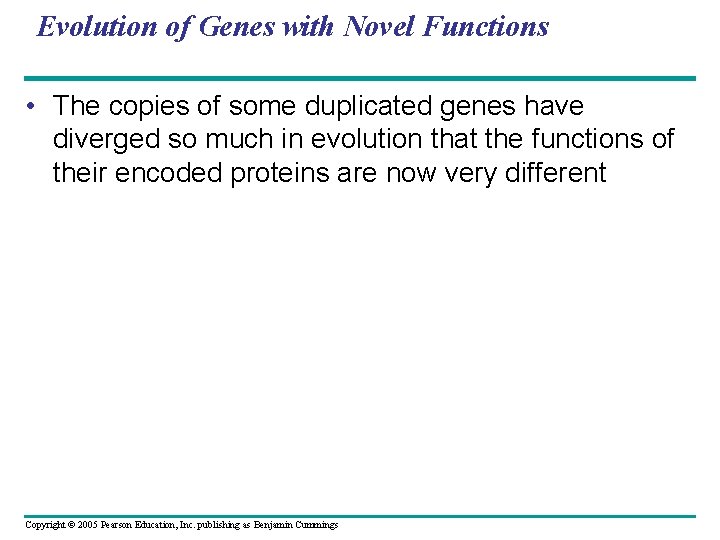
Evolution of Genes with Novel Functions • The copies of some duplicated genes have diverged so much in evolution that the functions of their encoded proteins are now very different Copyright © 2005 Pearson Education, Inc. publishing as Benjamin Cummings
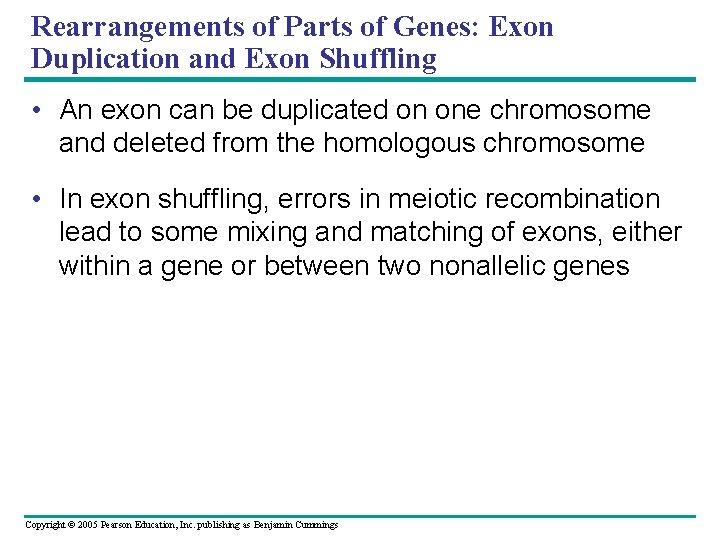
Rearrangements of Parts of Genes: Exon Duplication and Exon Shuffling • An exon can be duplicated on one chromosome and deleted from the homologous chromosome • In exon shuffling, errors in meiotic recombination lead to some mixing and matching of exons, either within a gene or between two nonallelic genes Copyright © 2005 Pearson Education, Inc. publishing as Benjamin Cummings
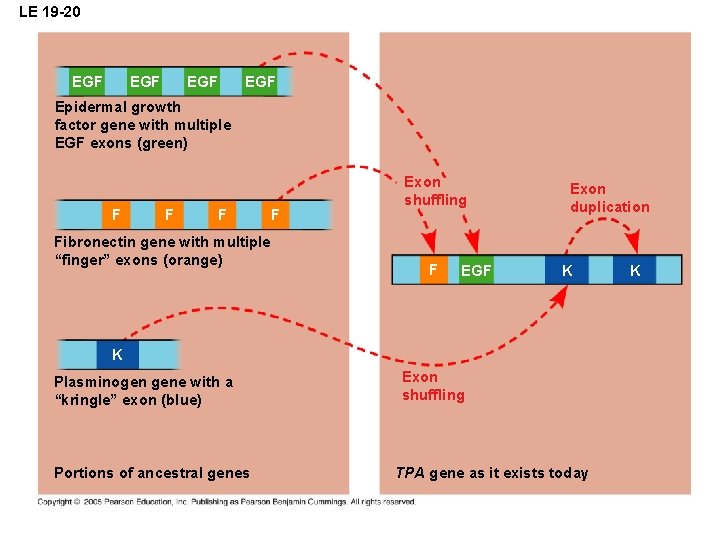
LE 19 -20 EGF EGF Epidermal growth factor gene with multiple EGF exons (green) F Fibronectin gene with multiple “finger” exons (orange) F Exon shuffling F EGF Exon duplication K K Plasminogen gene with a “kringle” exon (blue) Portions of ancestral genes Exon shuffling TPA gene as it exists today K
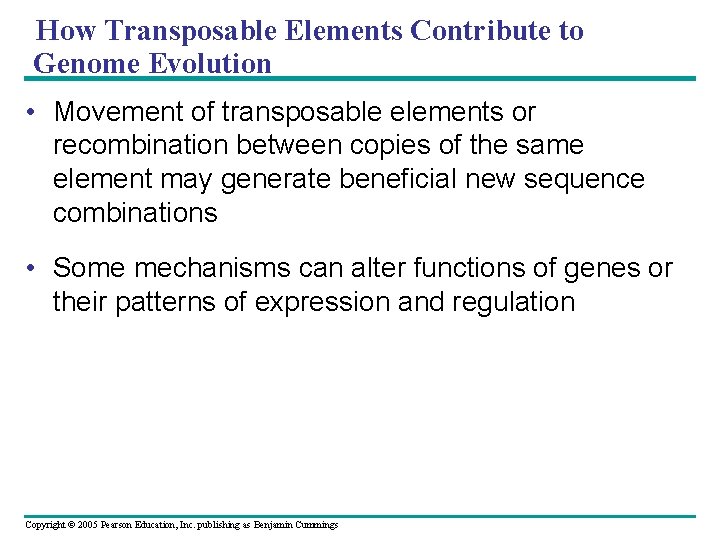
How Transposable Elements Contribute to Genome Evolution • Movement of transposable elements or recombination between copies of the same element may generate beneficial new sequence combinations • Some mechanisms can alter functions of genes or their patterns of expression and regulation Copyright © 2005 Pearson Education, Inc. publishing as Benjamin Cummings
Chapter 18 genomes and their evolution
Computational biology: genomes, networks, evolution
Block organization essay- examples
Chapter 12 section 4 gene regulation and mutations
Process organization in computer organization
Chapter 18 regulation of gene expression
Chapter 18 regulation of gene expression
Chapter 18 regulation of gene expression
Ch 18
Evolution and community ecology guided notes
Chapter 21 section 1 plant evolution and adaptations
Chapter 5 evolution and community ecology
Chapter 5 evolution and community ecology answer key
Chapter 4 biodiversity and evolution
Anthocerophytes
Chapter 24 animal evolution diversity and behavior
Chapter 14 evolution a history and a process
Amoebozoa definition
Multicellular heterotrophic
Prokaryotes vs eukaryotes venn diagram
Prokaryotic and eukaryotic cells
Eukaryotic and aerobic
Venn diagram of plants and animals
Three parts of cell theory
Cytoskeletal protein
Cytoskeleton prokaryotic or eukaryotic
What is eukaryotic and prokaryotic
Similarity between prokaryotic and eukaryotic cells
Prokaryotic cell and eukaryotic cell
Answers
Prokaryotic and eukaryotic cells chart
Prokaryotic vs eukaryotic
Difference between prokaryotic and eukaryotic cell
How water moves
Differences between prokaryotic and eukaryotic cells
Anatomy of prokaryotes and eukaryotes
What is thermoregulation
Section 4 gene regulation and mutations
Table tennis regulations
Homeostasis mechanisms for regulation of body temperature
Recyclability and self regulation in nature
Three stages of glycolysis
Nco authority
Regulation of recruitment and placement activities
Table tennis rules and regulation
Regulation and inspection of social care wales
Government regulation and the legal environment of business
Hines hill train crash
Michigan department of licensing and regulation
Licensing and regulation division
Osha hazard and ghs training regulation cfr 1910
Ground water management and regulation scheme
Blood bank regulation under drugs and cosmetics act
Chapter 5 how to form a business
Strategic management chapter 3
Organizational culture diagnosis worksheet
Chapter 16 evolution of populations vocabulary review
Chapter 17 evolution of populations answer key
Strengths and weaknesses of evolutionary theory page 386
Chapter 7 the evolution of living things answers
Chapter 23: the evolution of populations
Chapter 18 the evolution of invertebrate diversity
Chapter 17 darwin's theory of evolution
Chapter 16 section 1 primates
Chapter 16 evolution of populations
Chapter 15 darwin's theory of evolution section 15-1
Homologous structures definition biology
Chapter 6 the evolution of exteriors
Evolution of exteriors
Chapter 23 the evolution of populations
The evolution of management theory
Chapter 16 primate evolution
Molecular biology evidence of evolution
Pseudocde
Advanced evolution chapter 4
Advanced part modeling
Natural selection
Chapter 7 the evolution of living things answers
Is a venus fly trap prokaryotic or eukaryotic
Most prominent organelle in eukaryotic cells
Eukaryotic cells
Prokaryotic cell vs eukaryotic cell
Plant cell
Parts of a prokaryotic cell