Chapter 12 Special Relativity and Elementary Particles Special
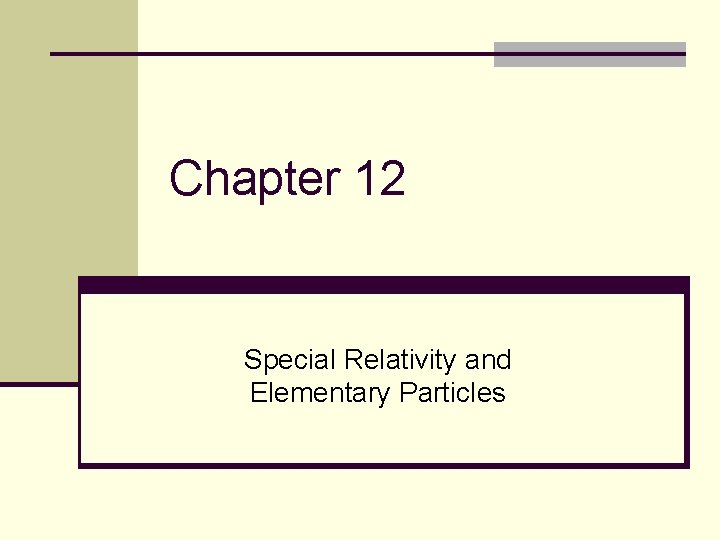
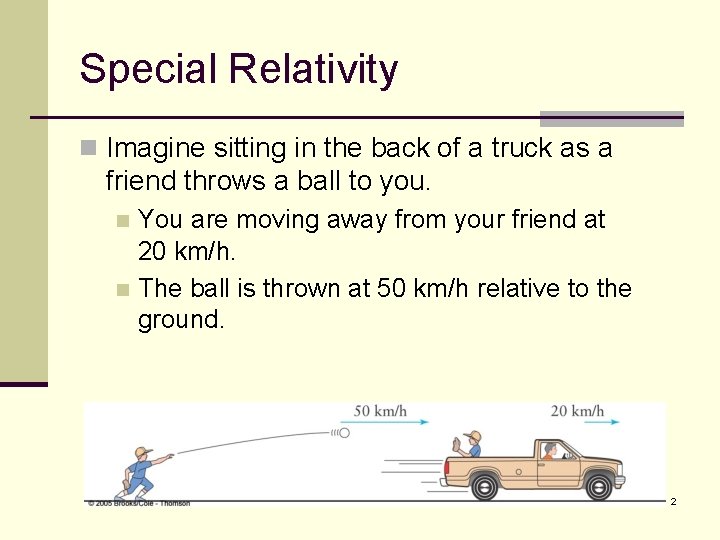
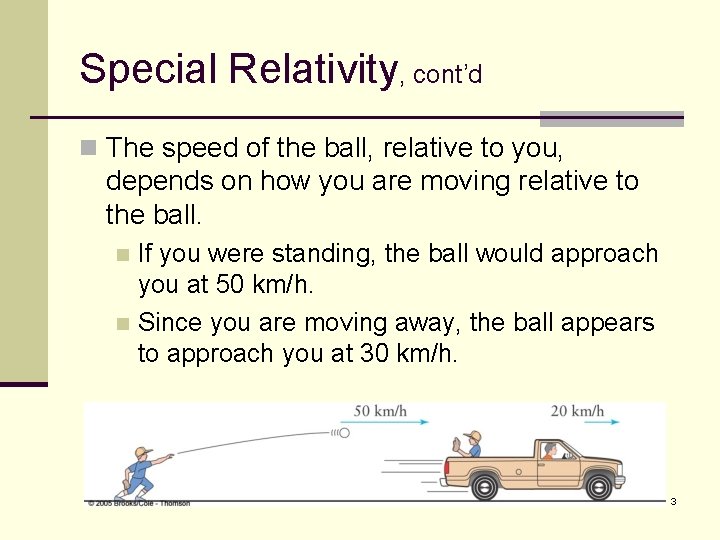
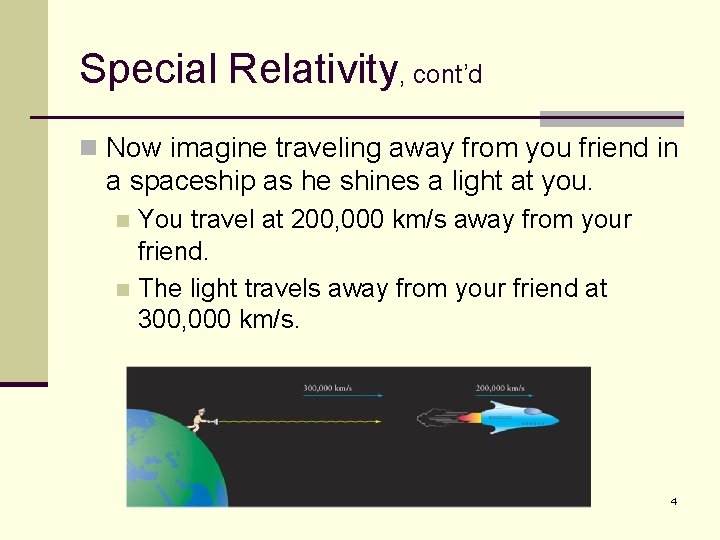
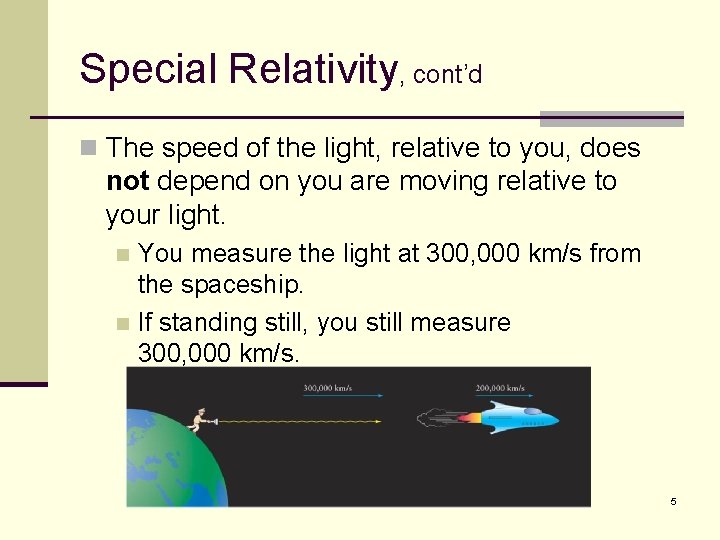
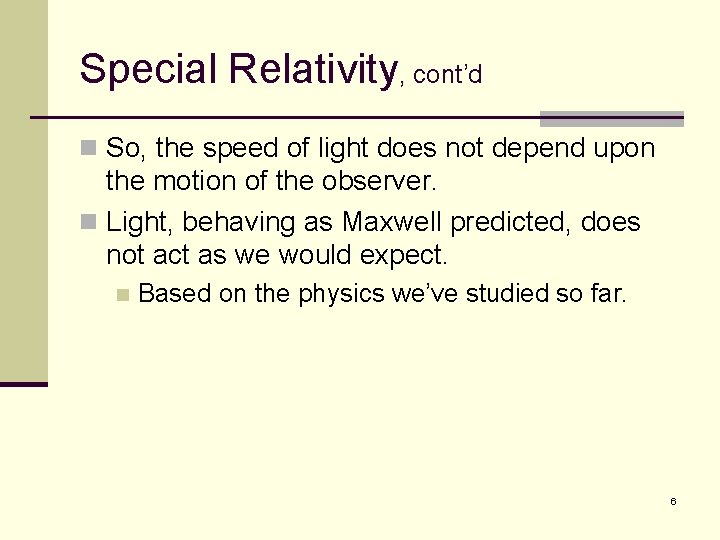
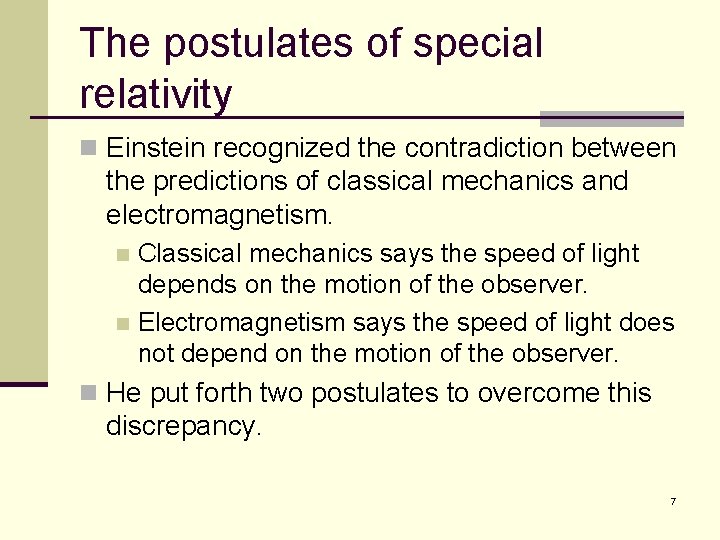
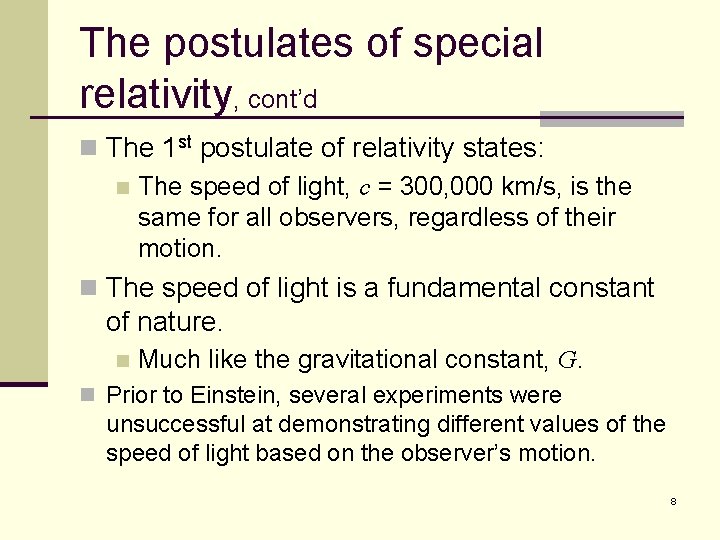
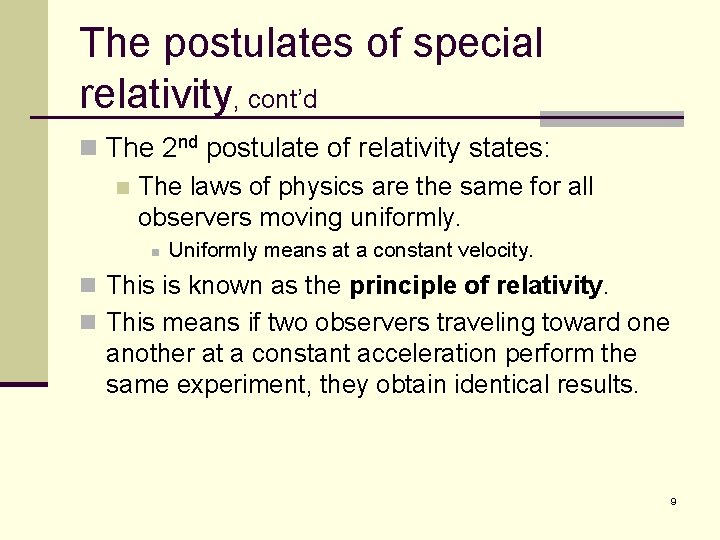
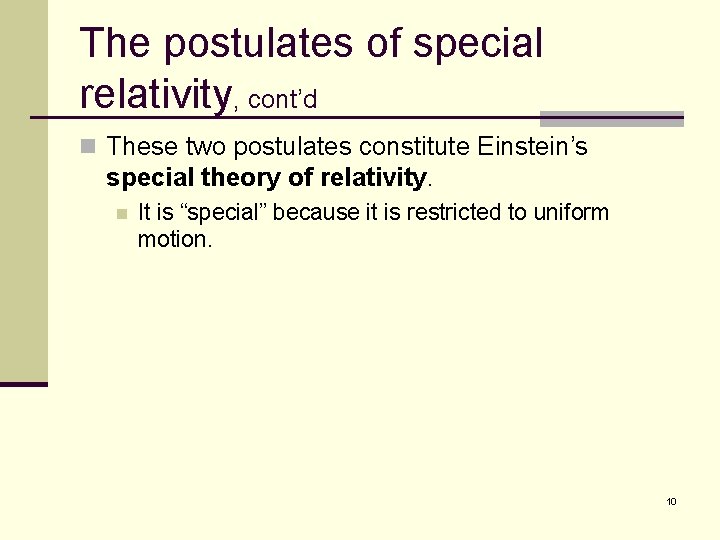
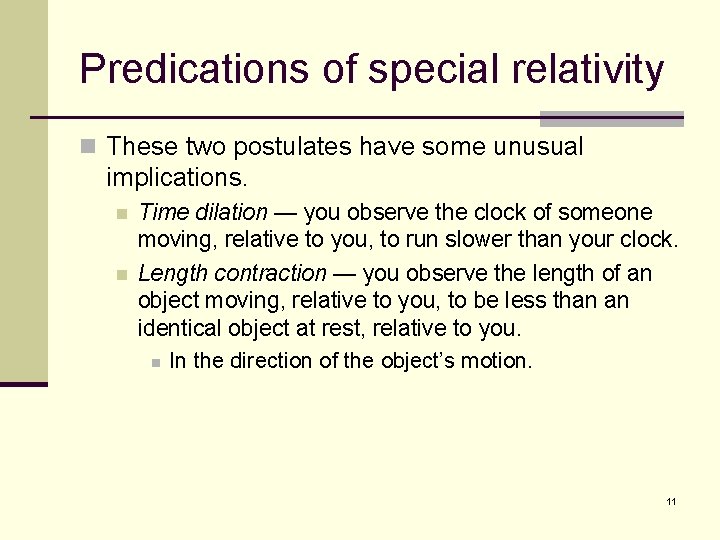
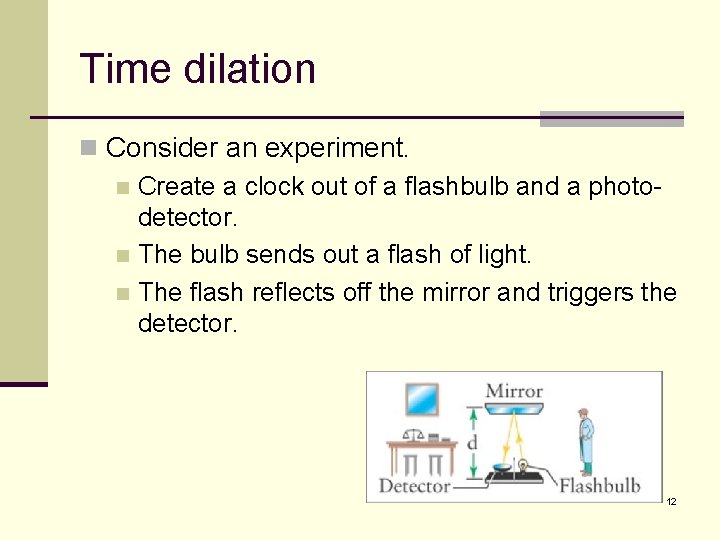
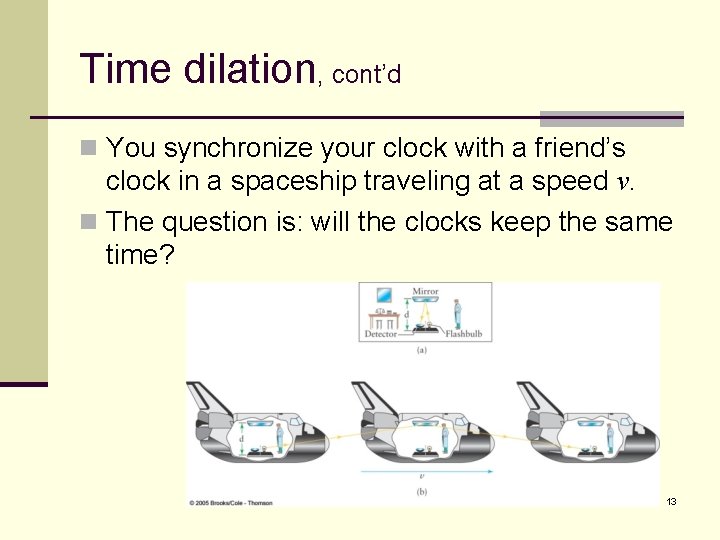
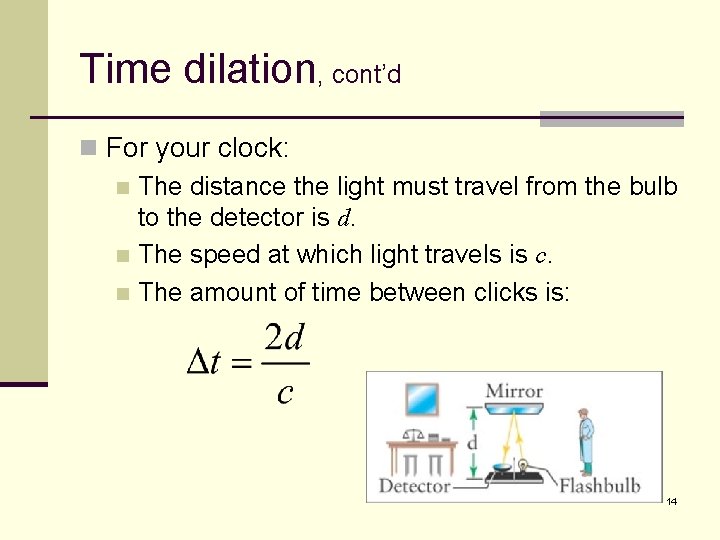
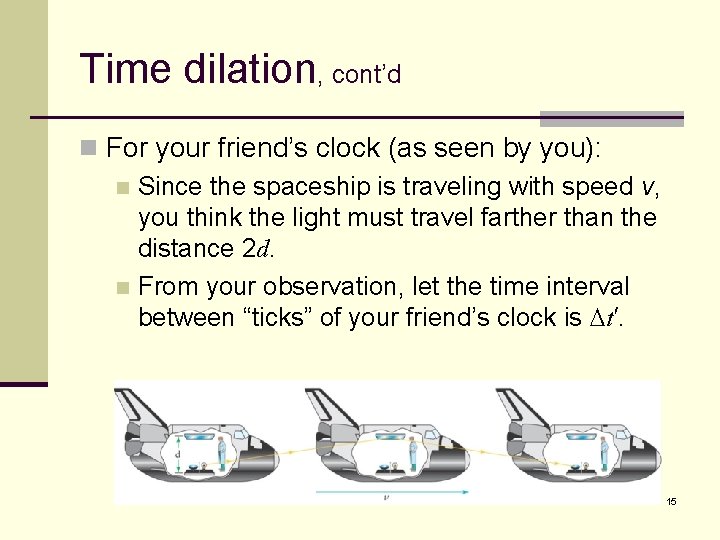
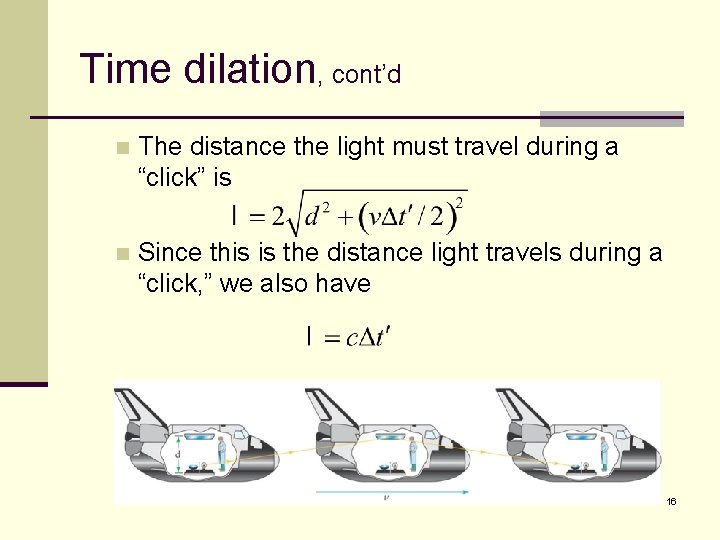
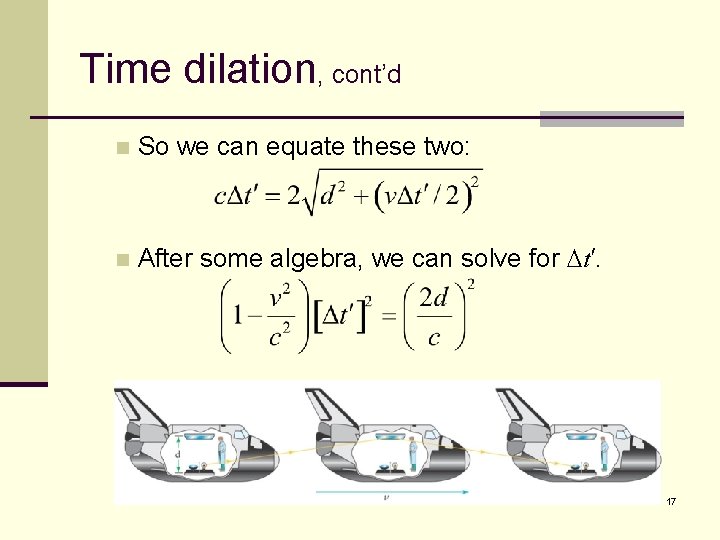
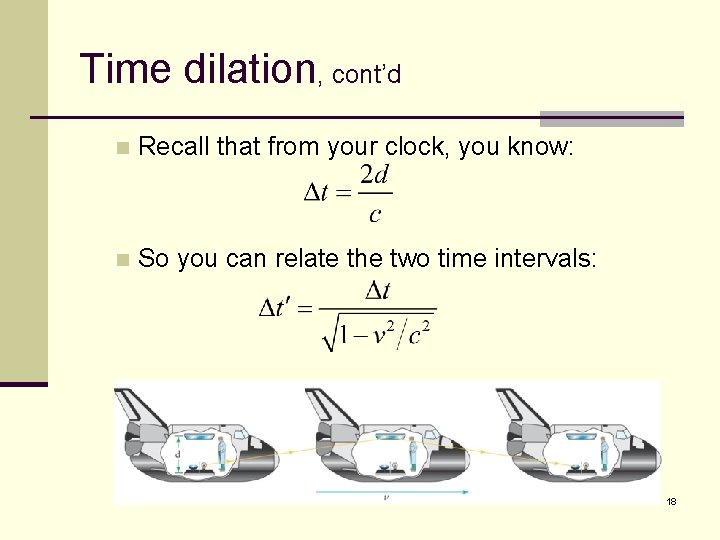
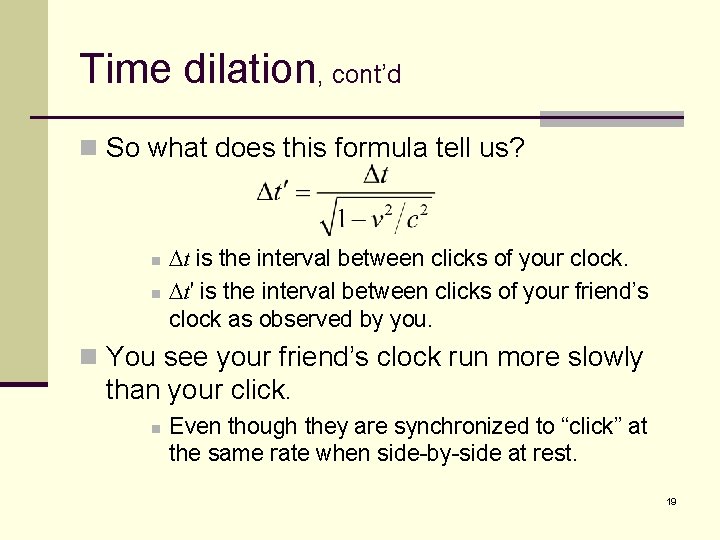
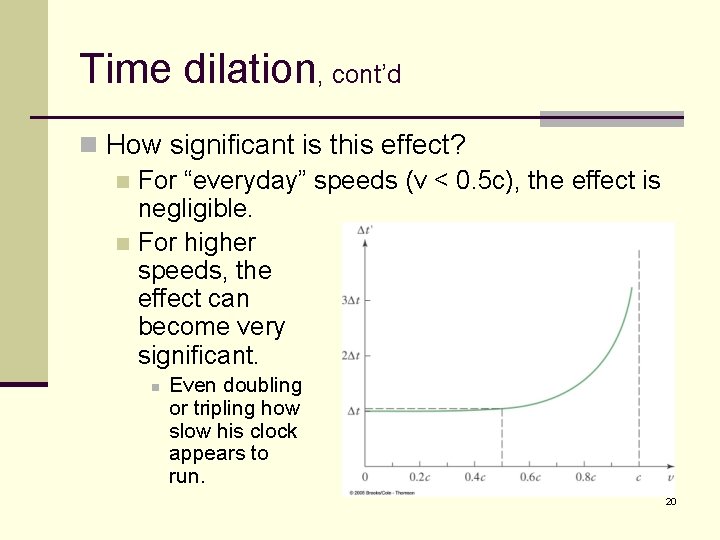
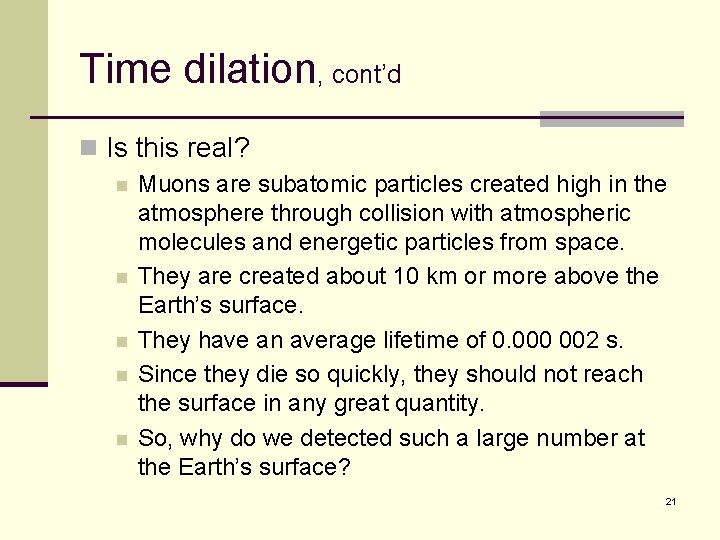
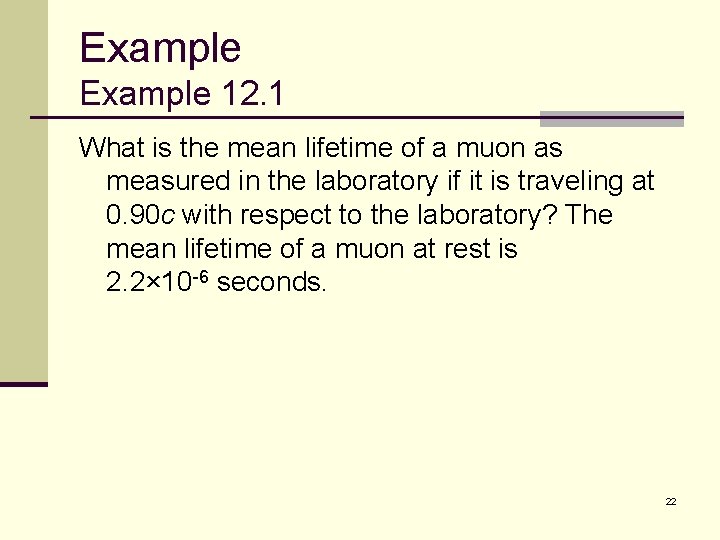
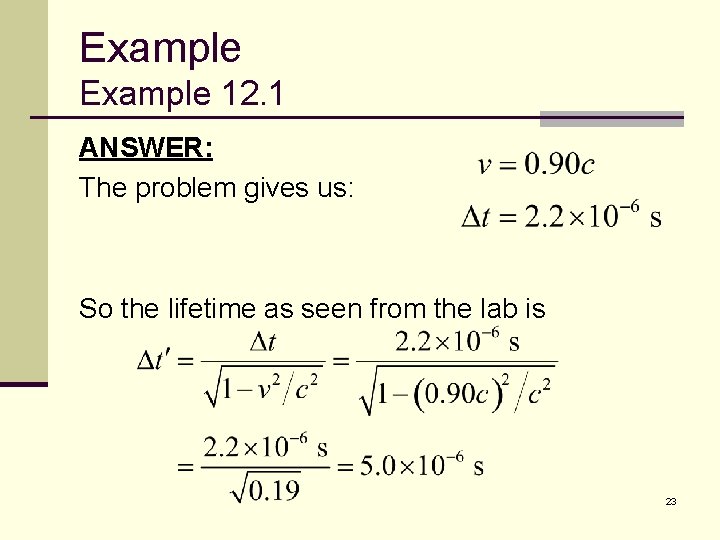
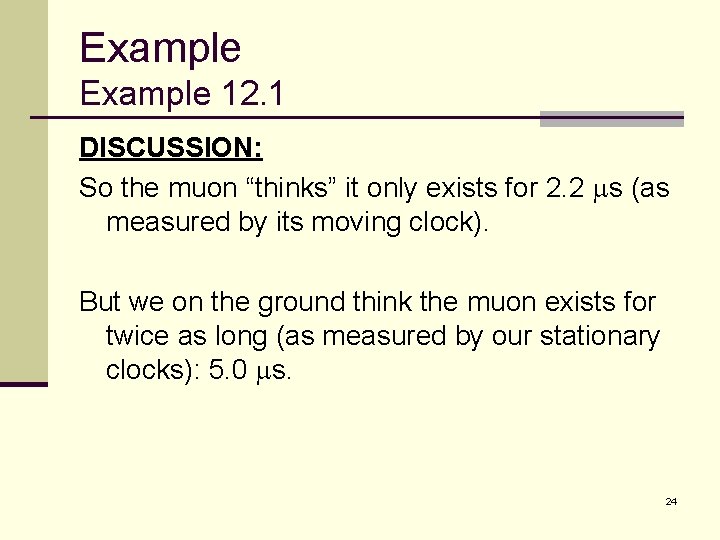
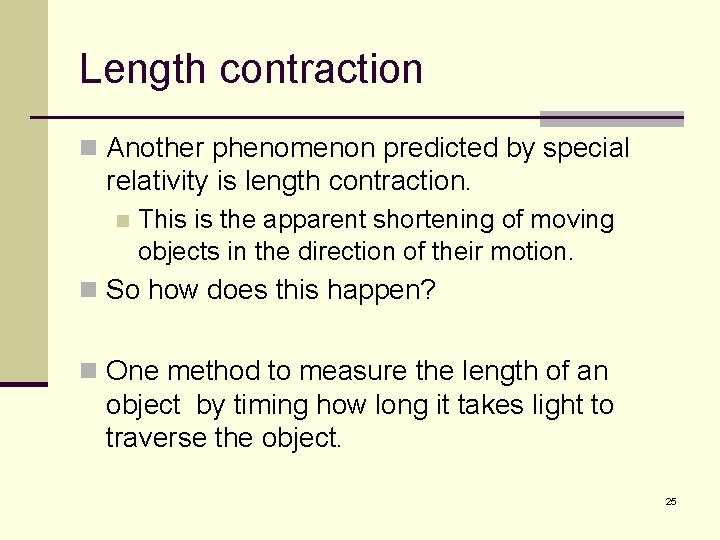
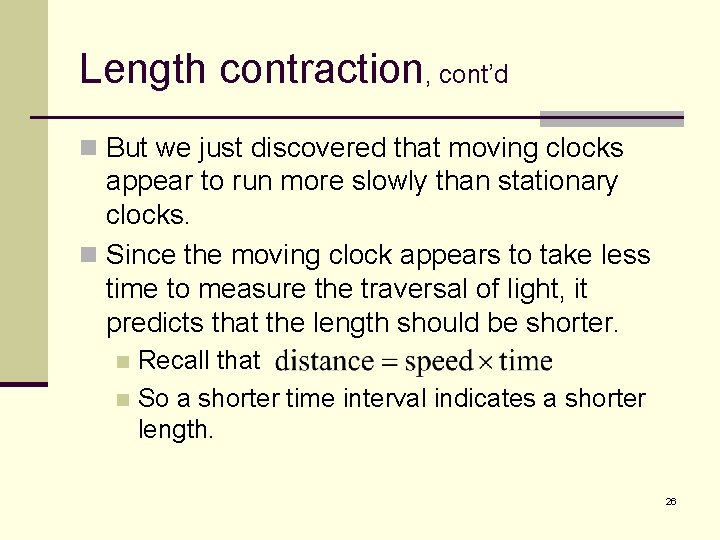
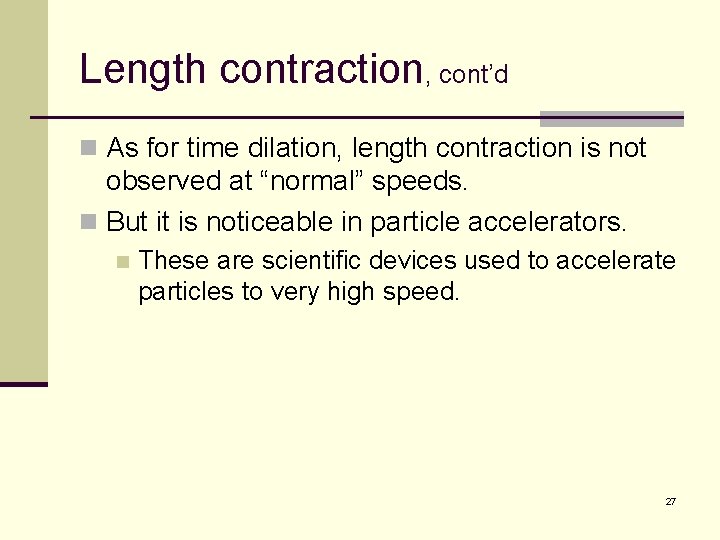
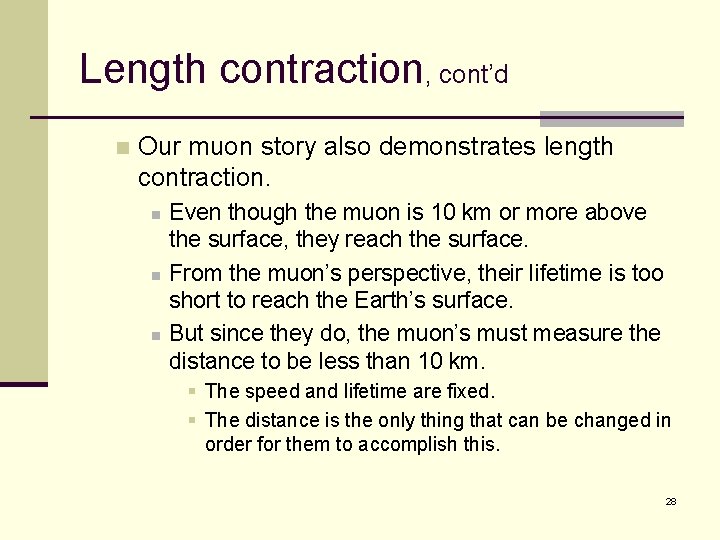
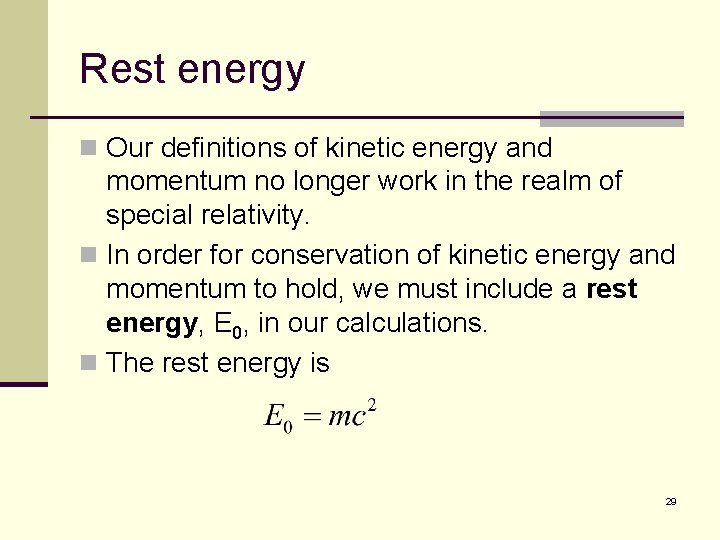
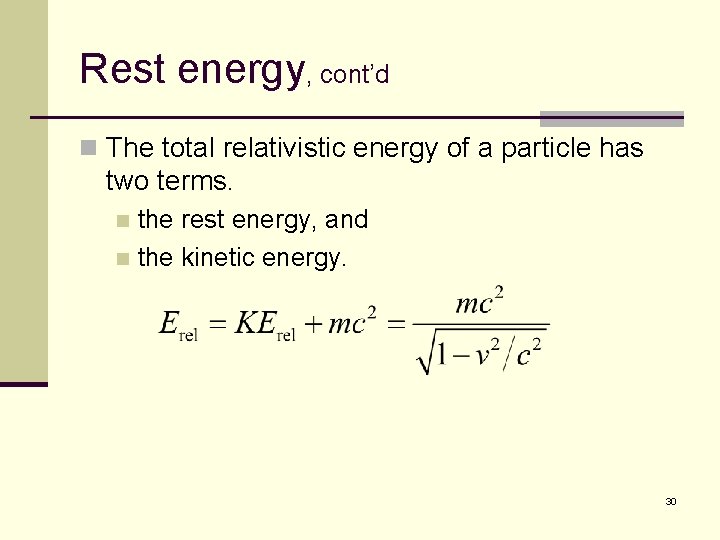
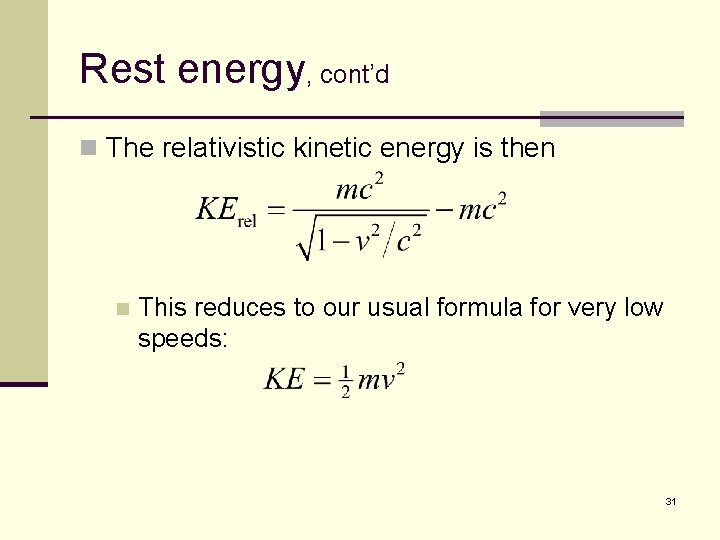
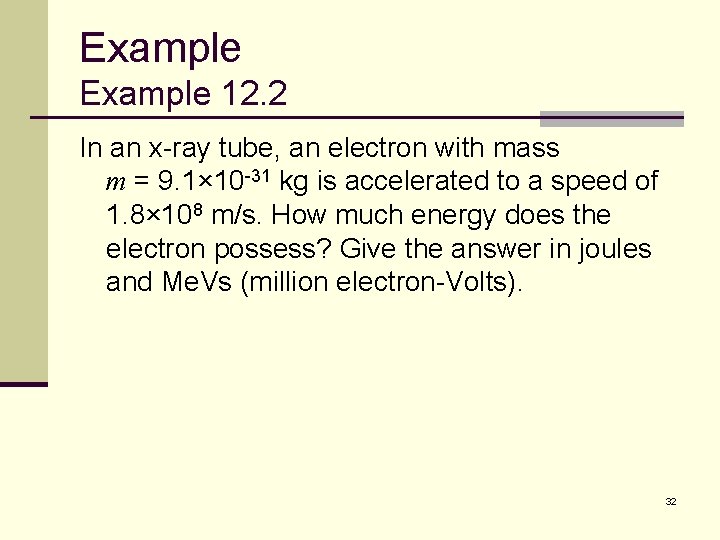
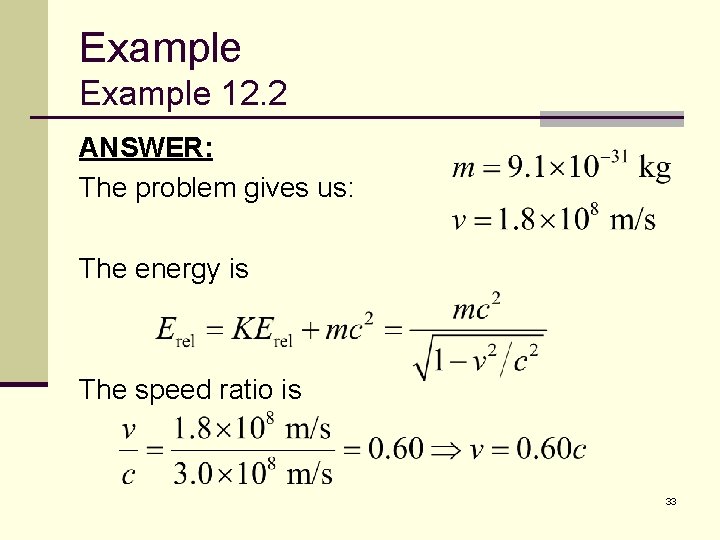
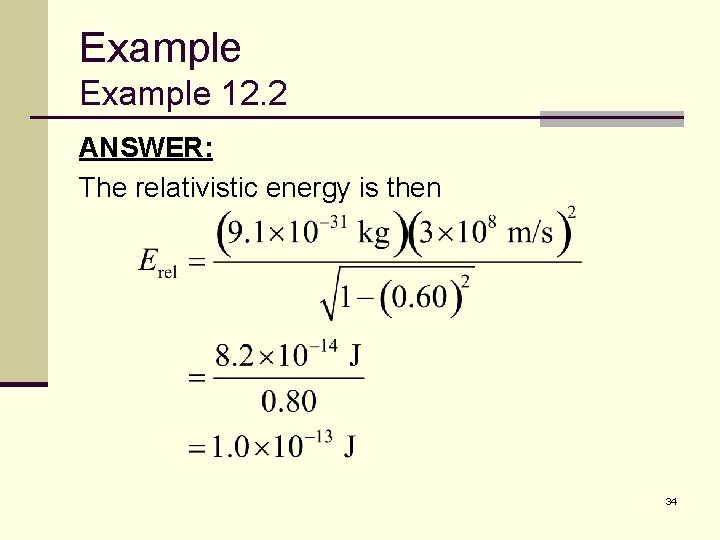
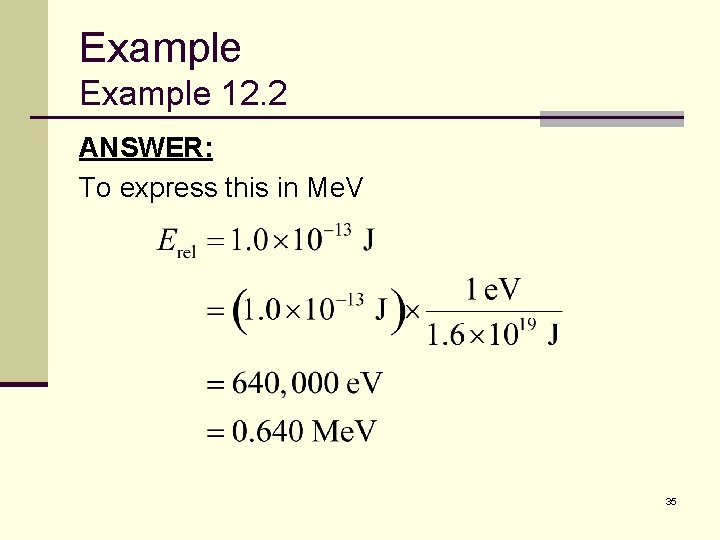
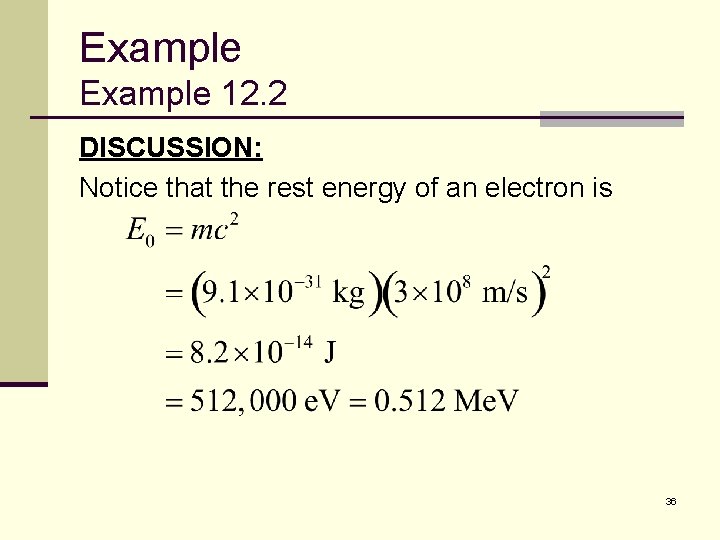
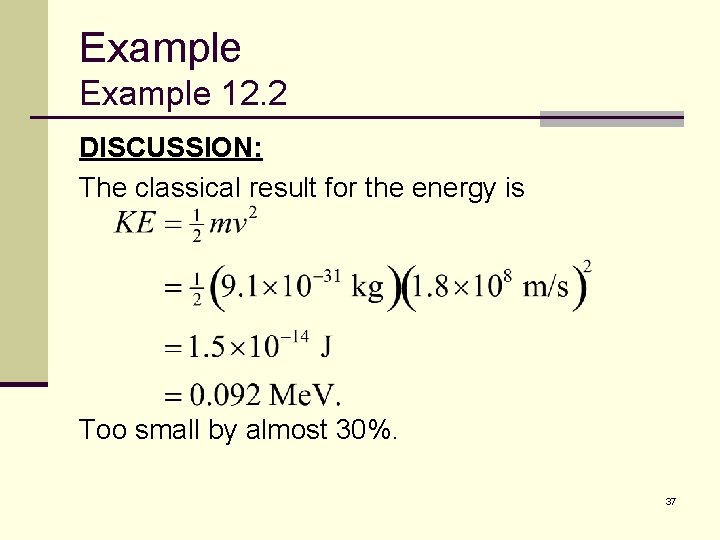
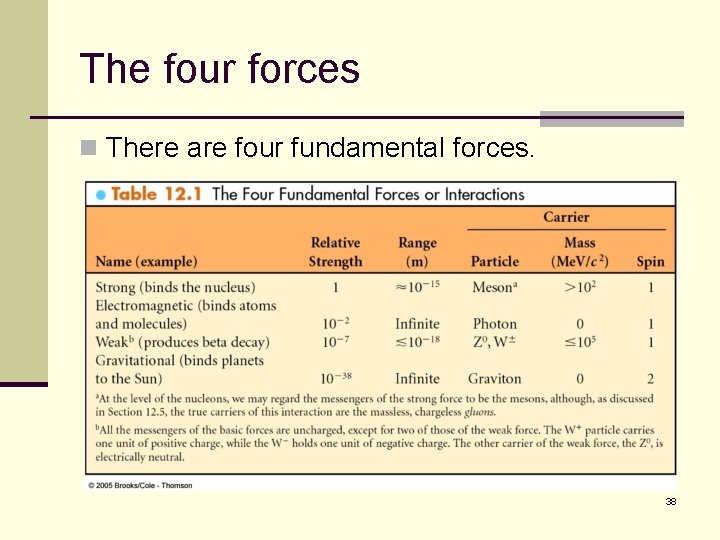
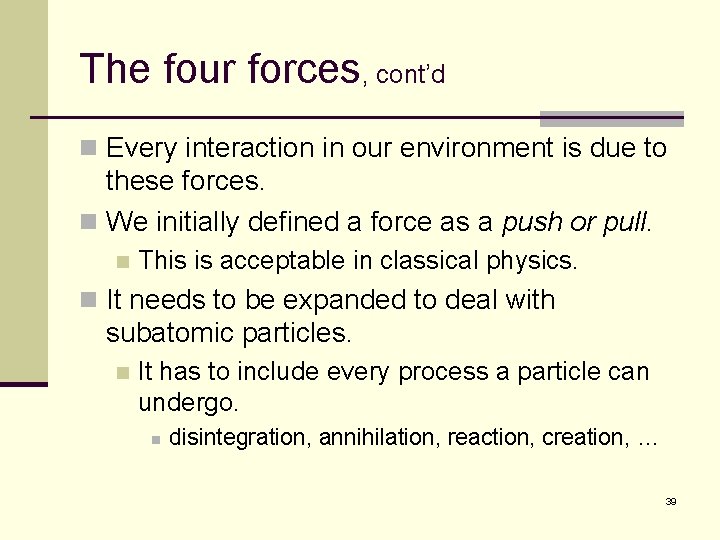
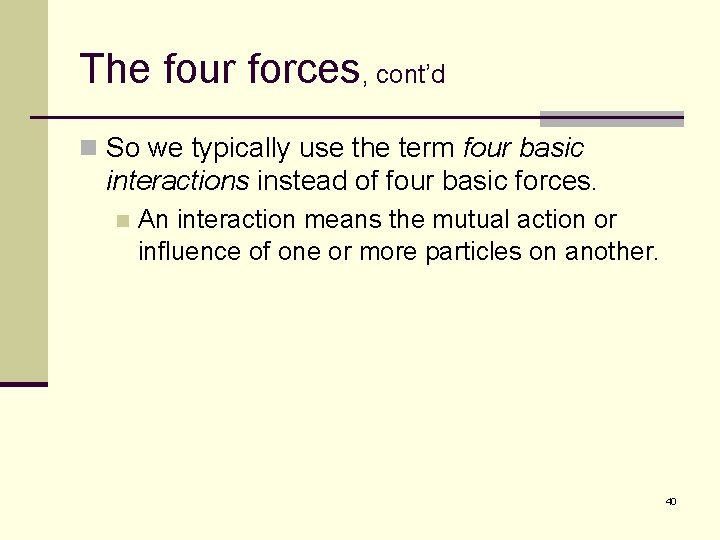
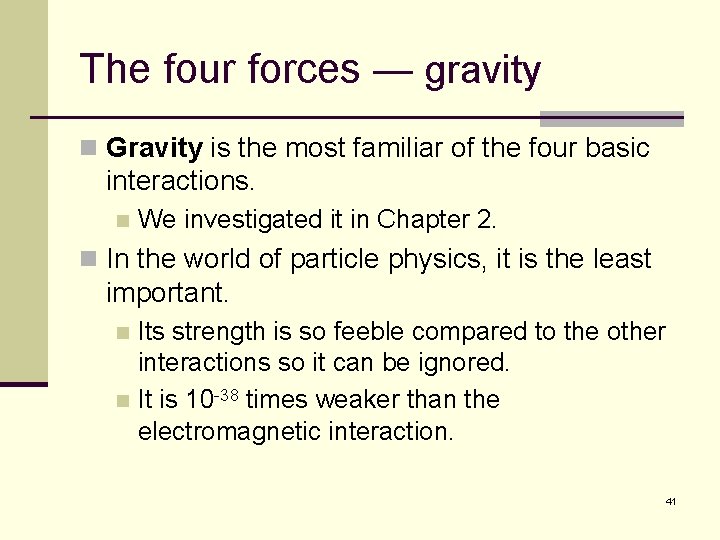
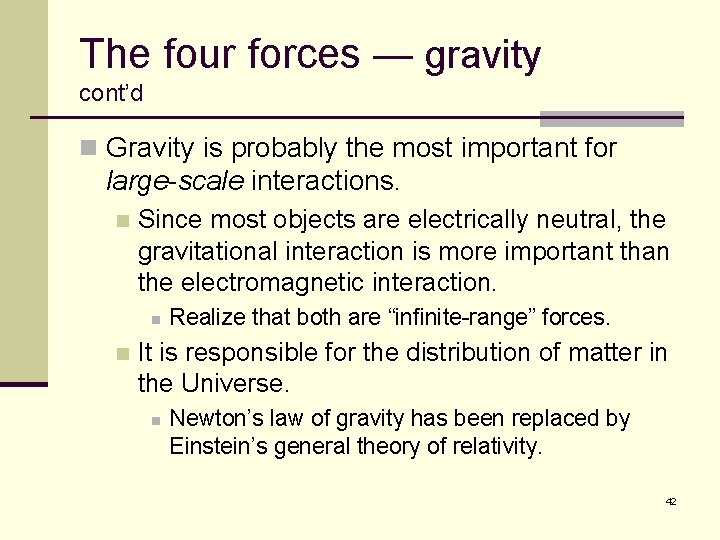
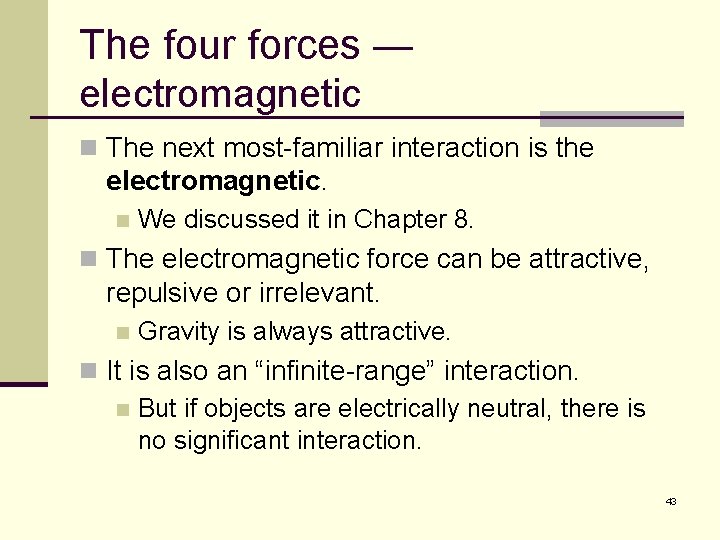
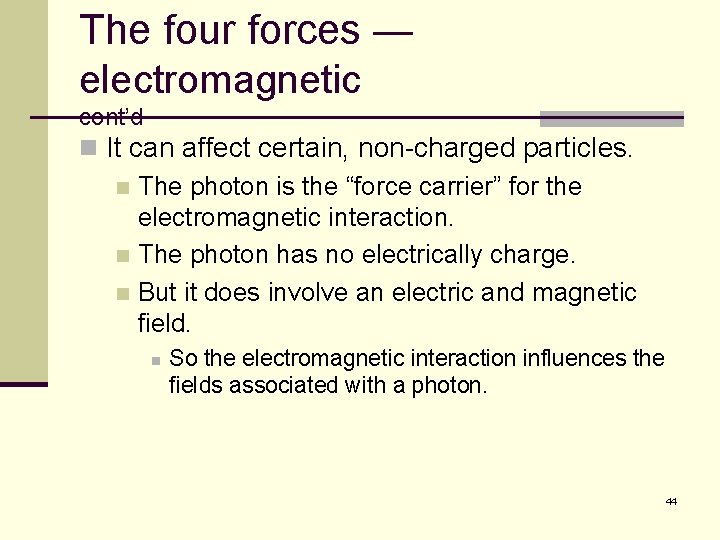
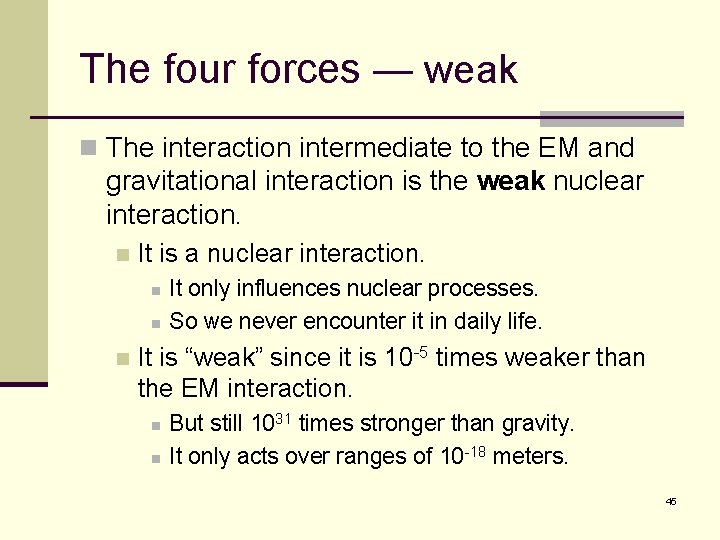
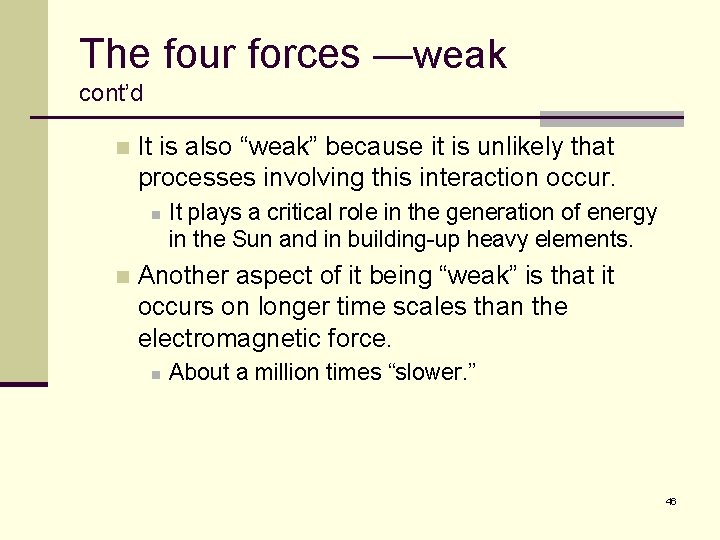
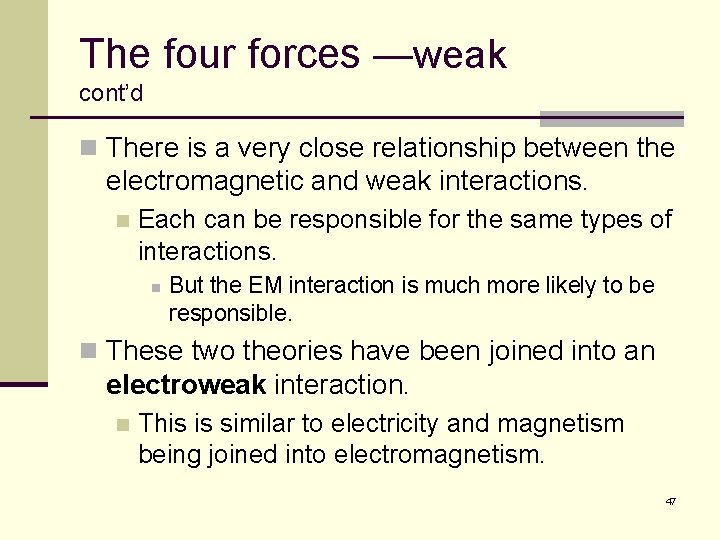
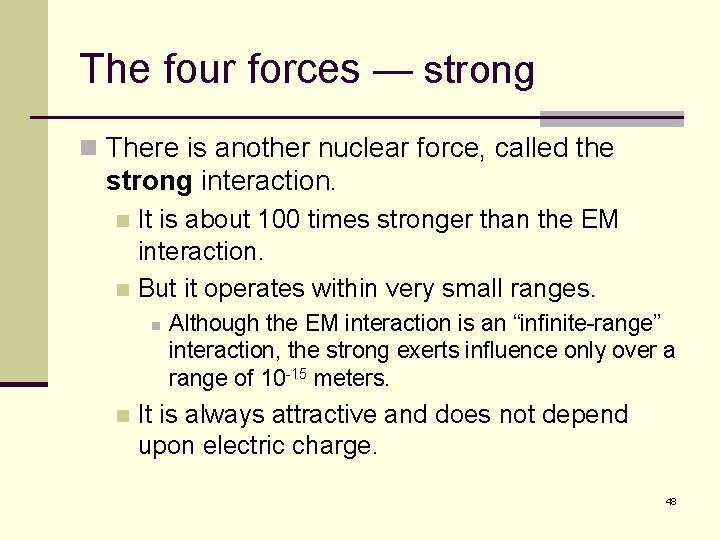
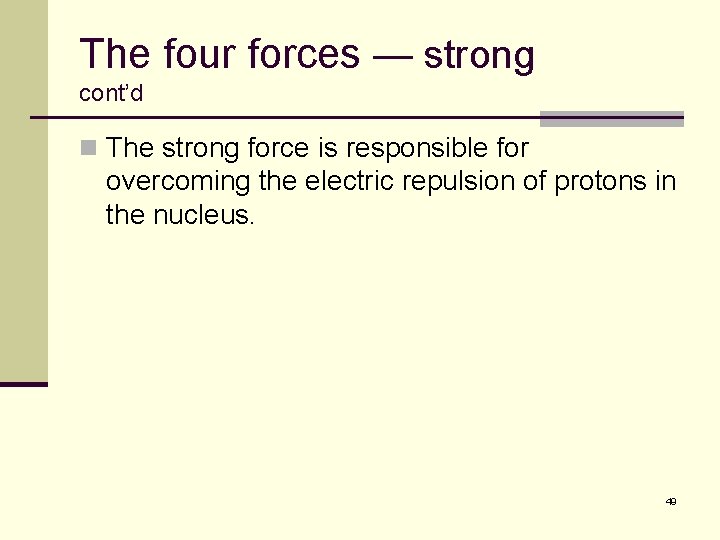
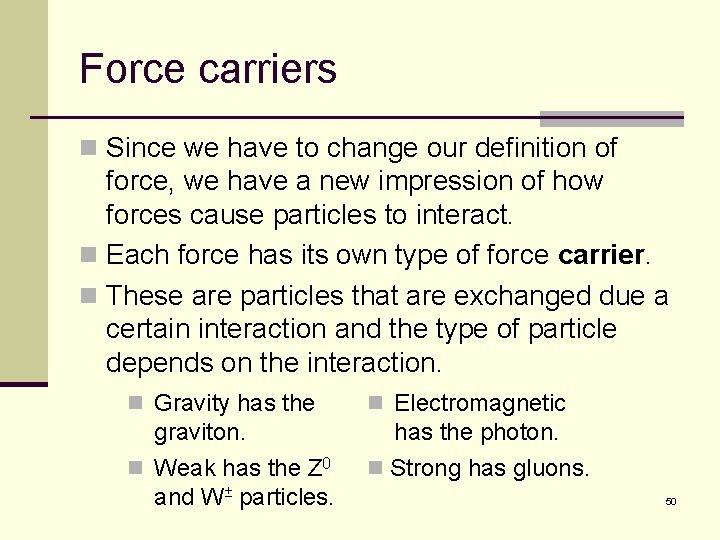
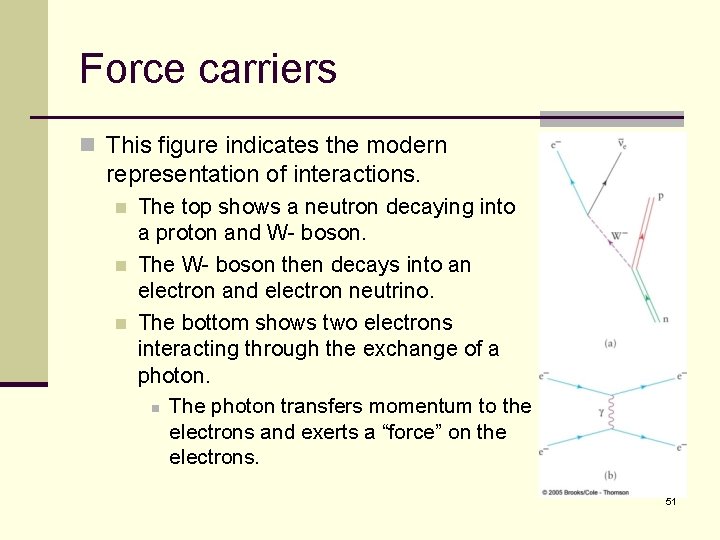
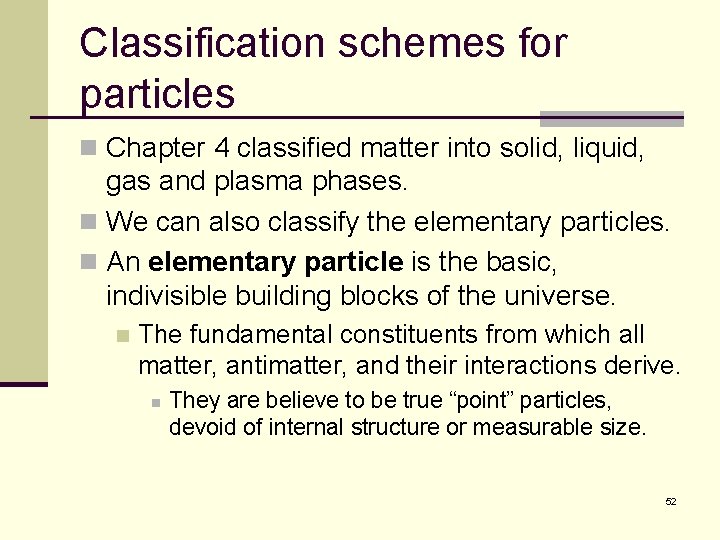
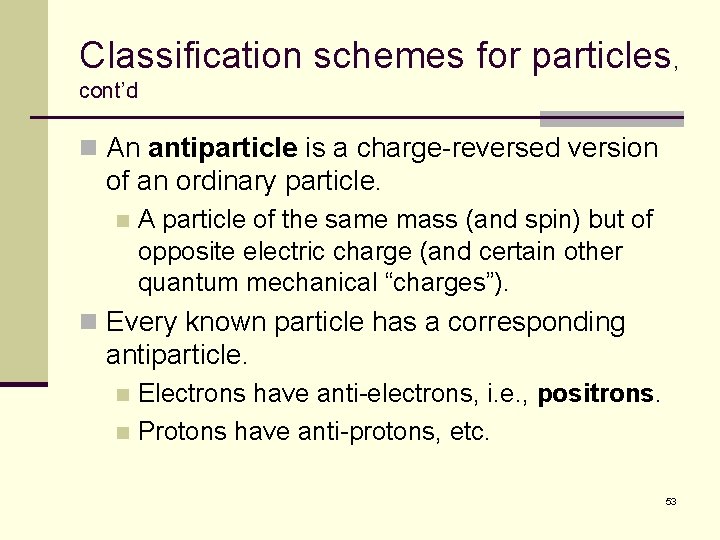
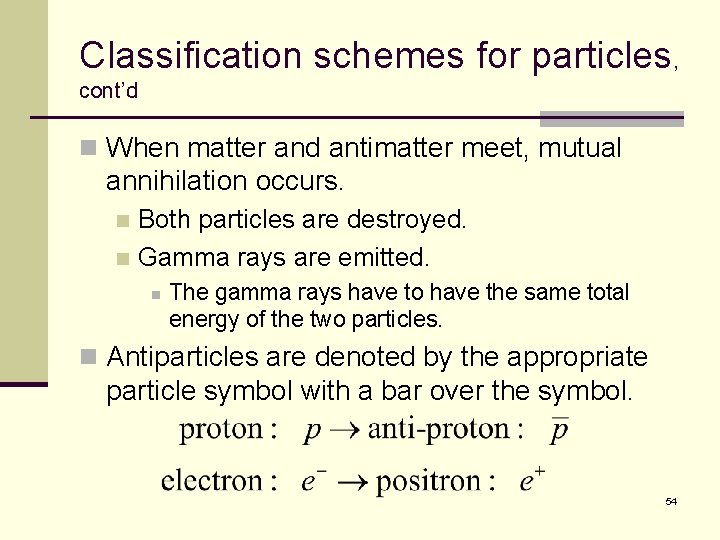
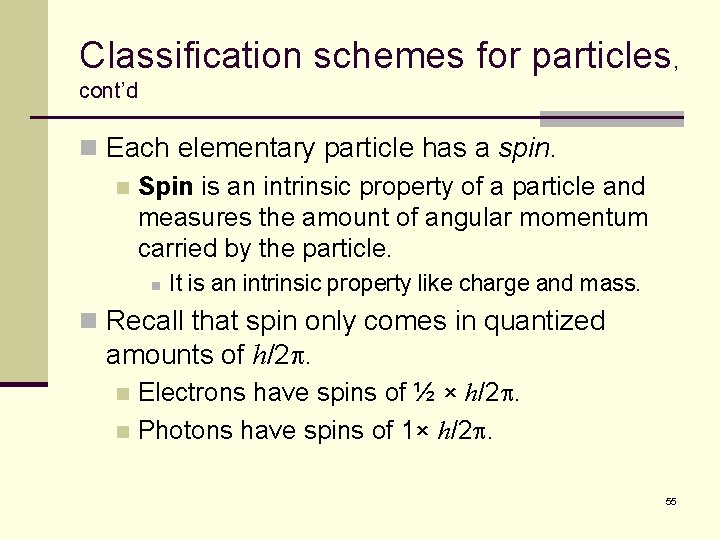
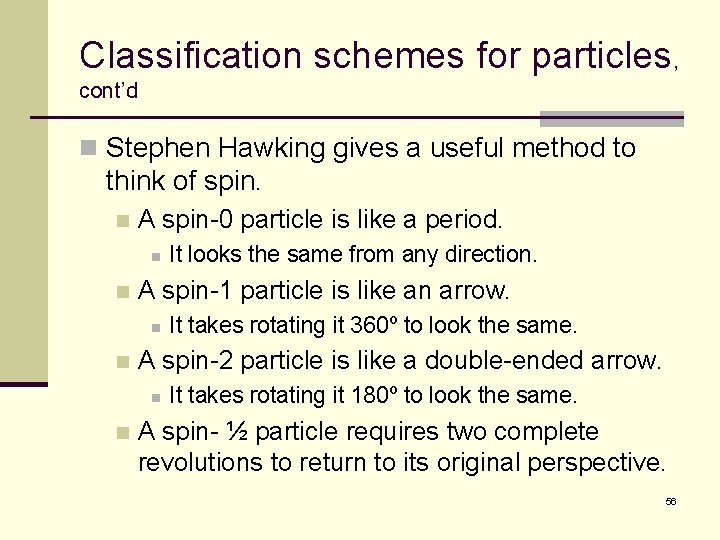
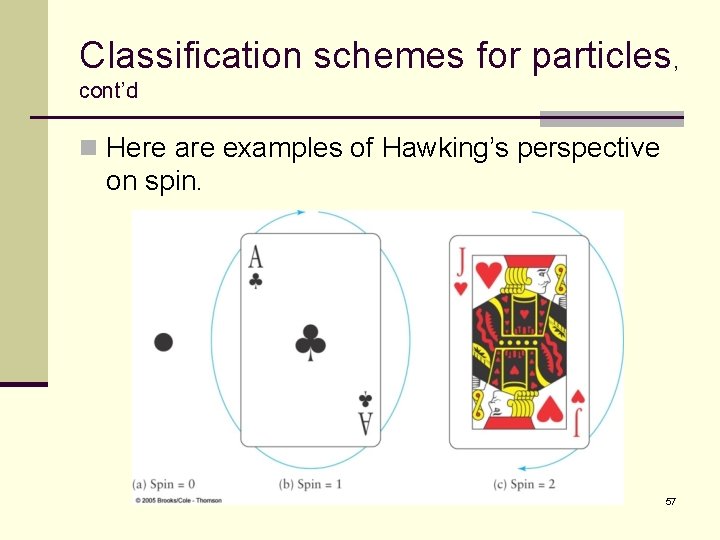
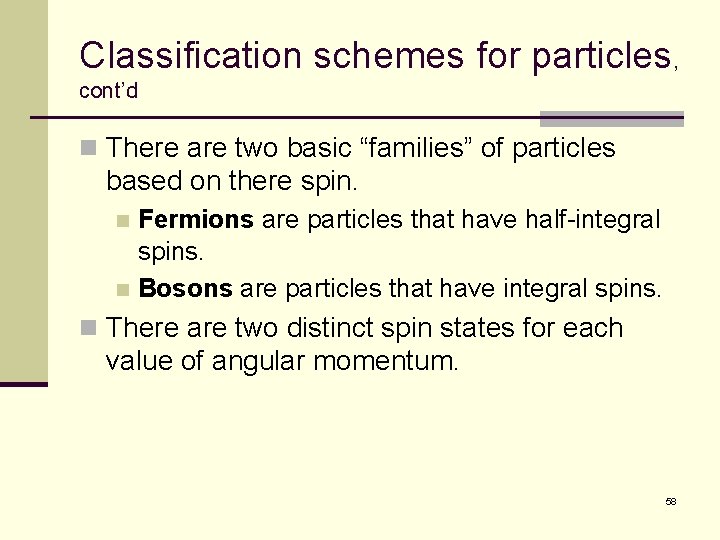
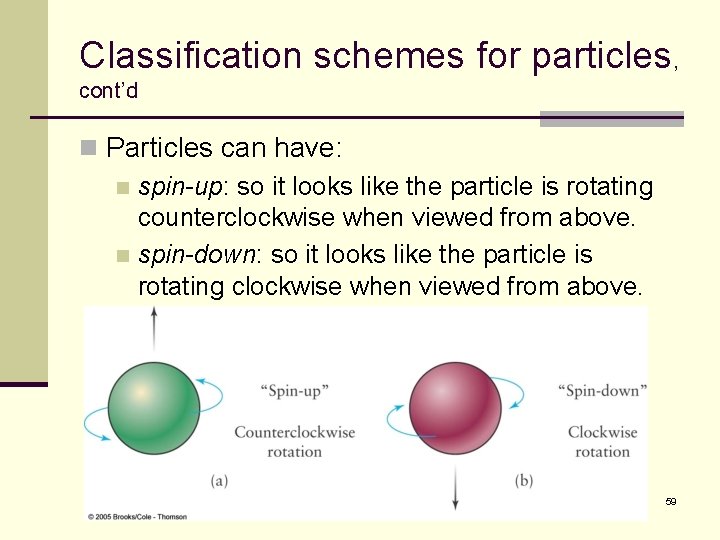
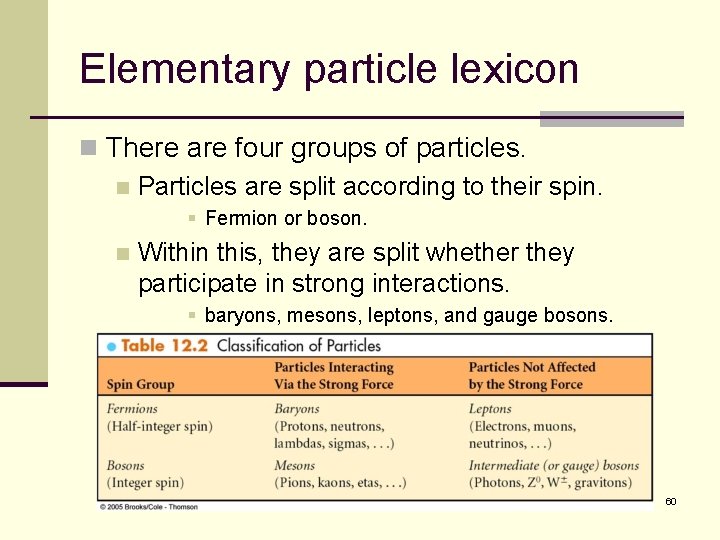
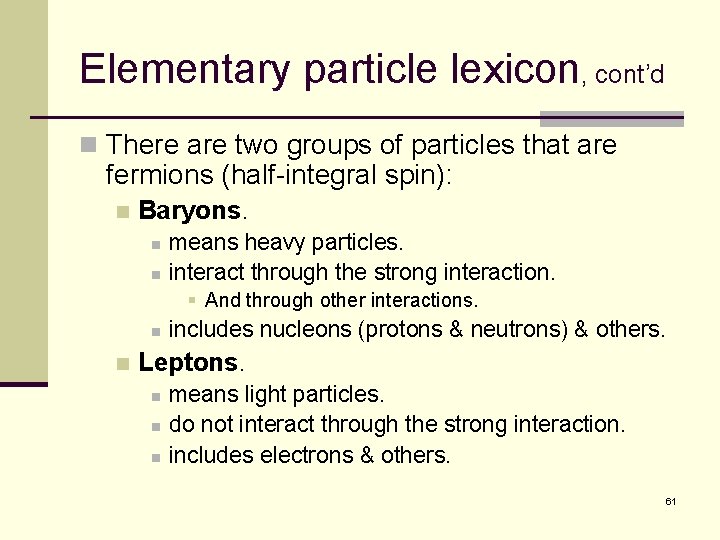
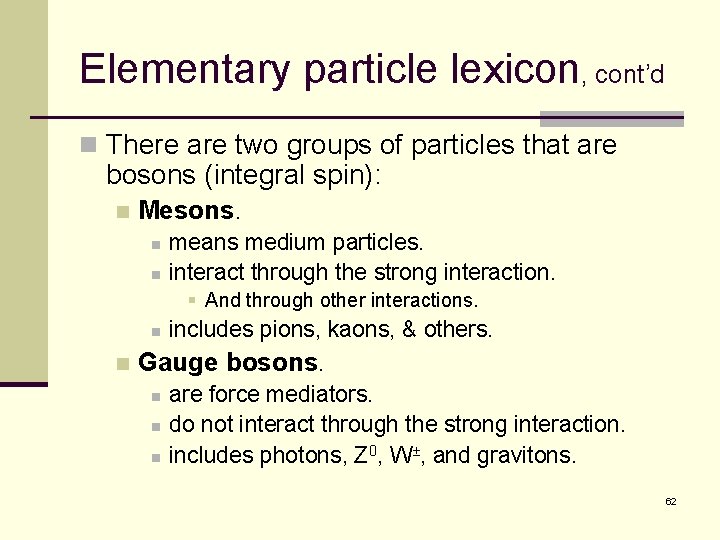
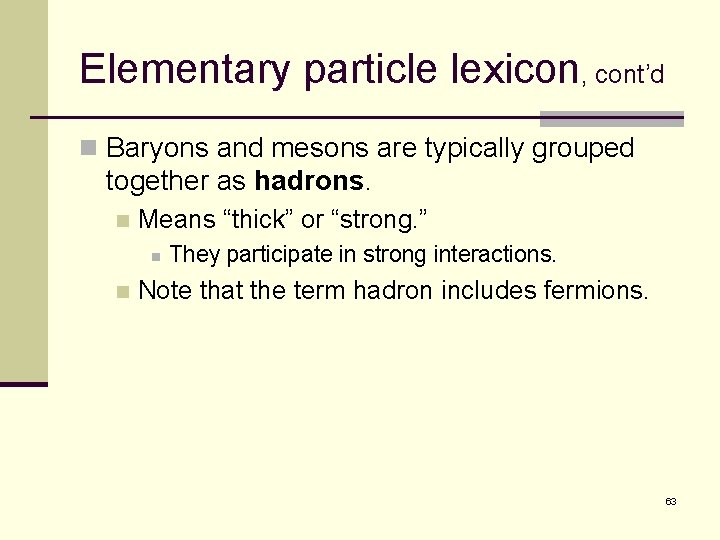
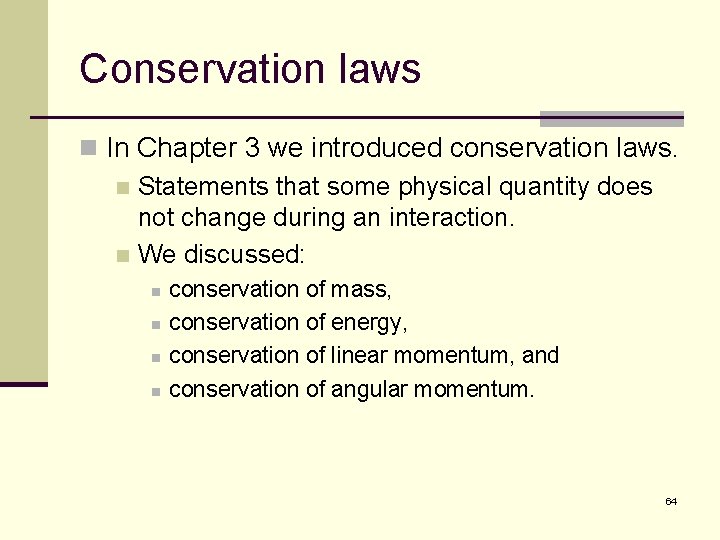
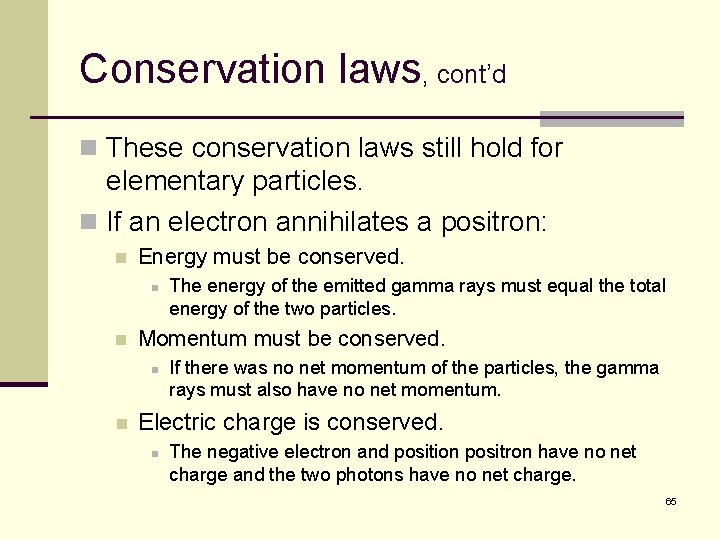
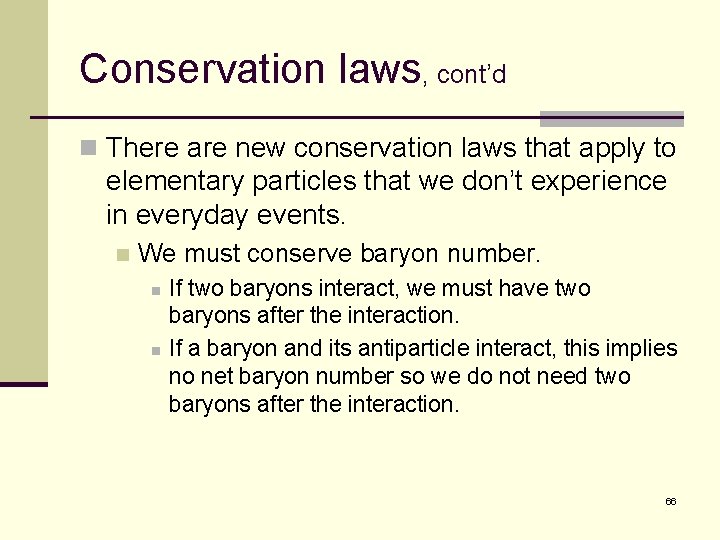
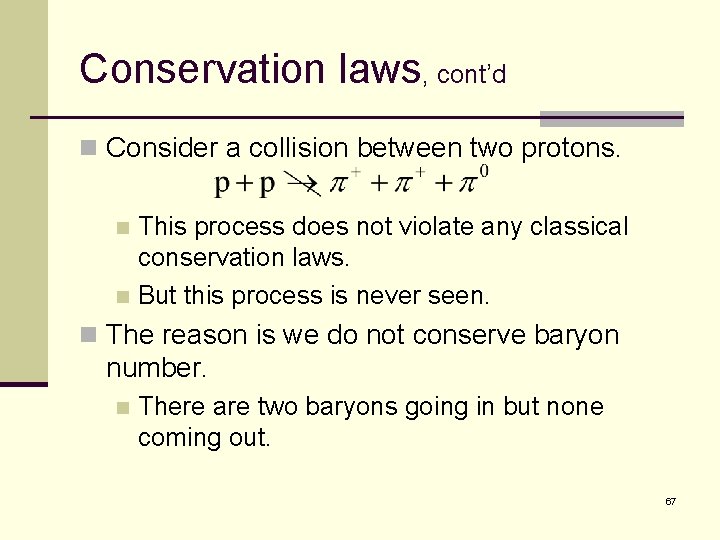
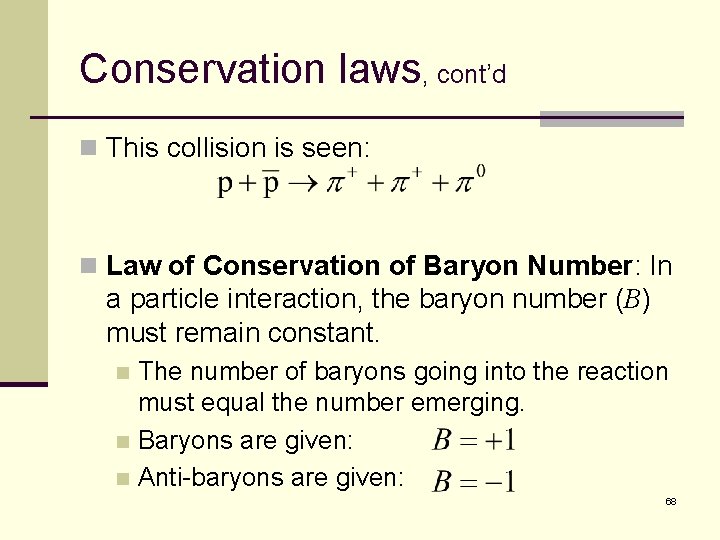
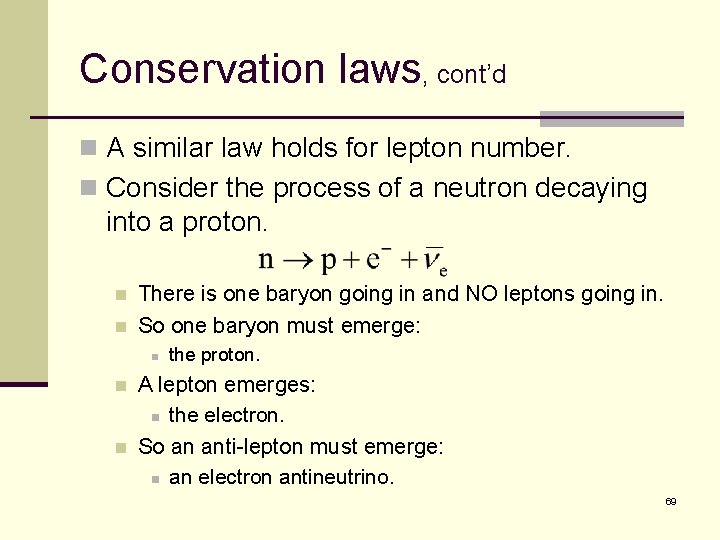
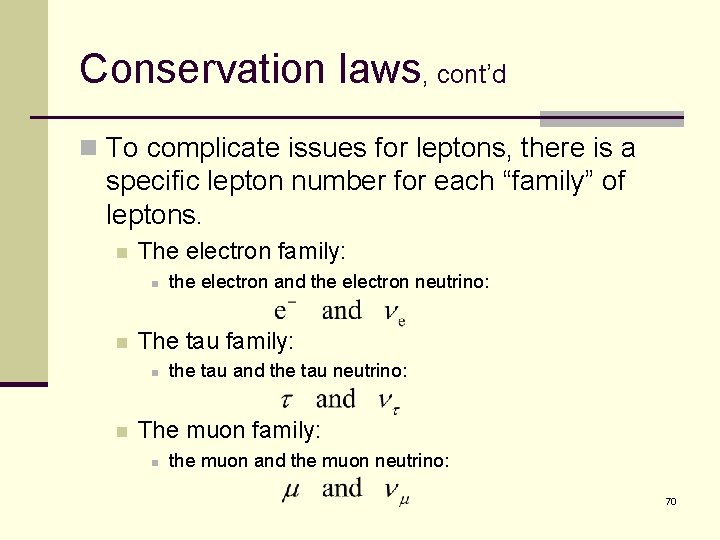
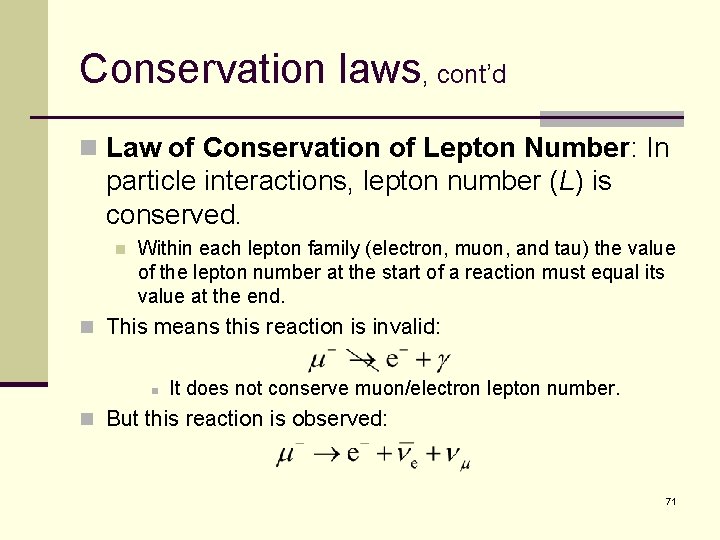
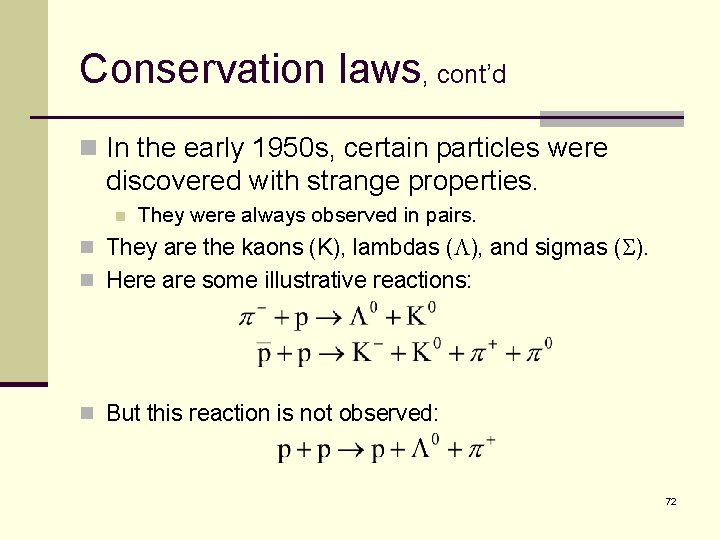
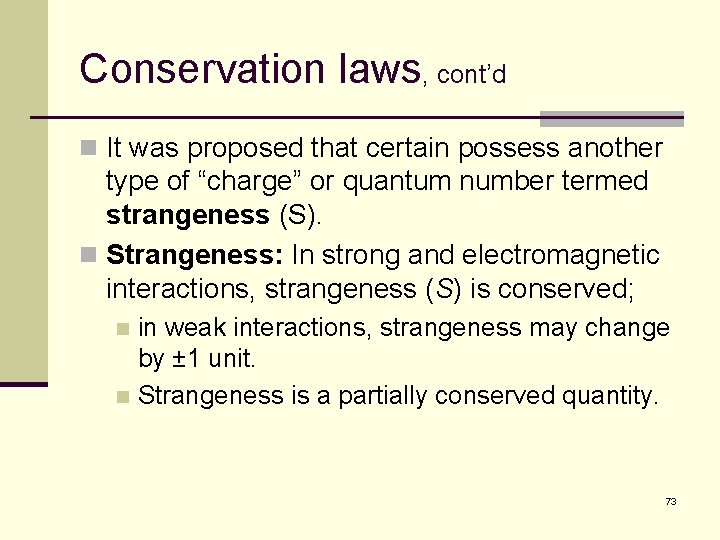
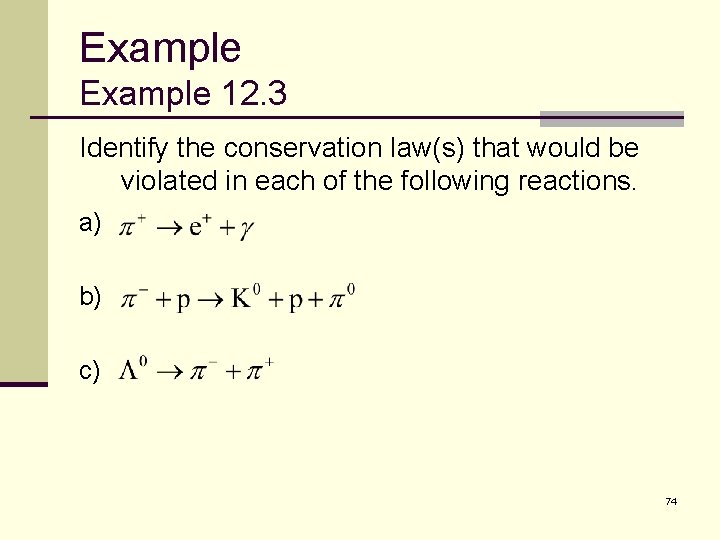
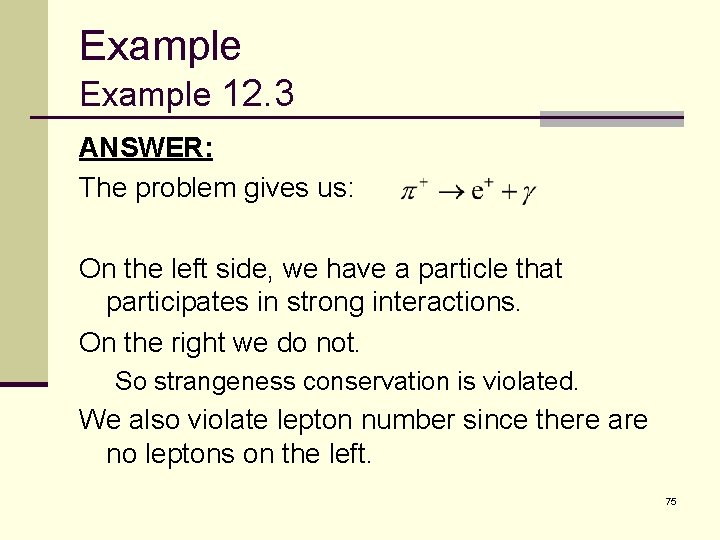
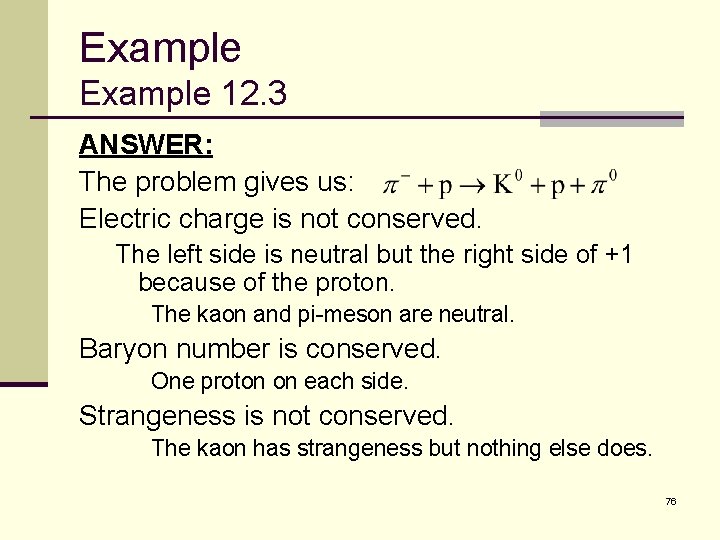
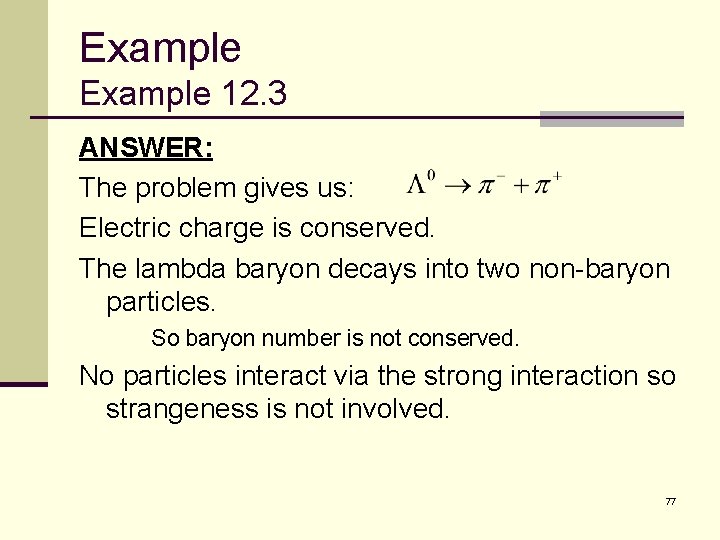
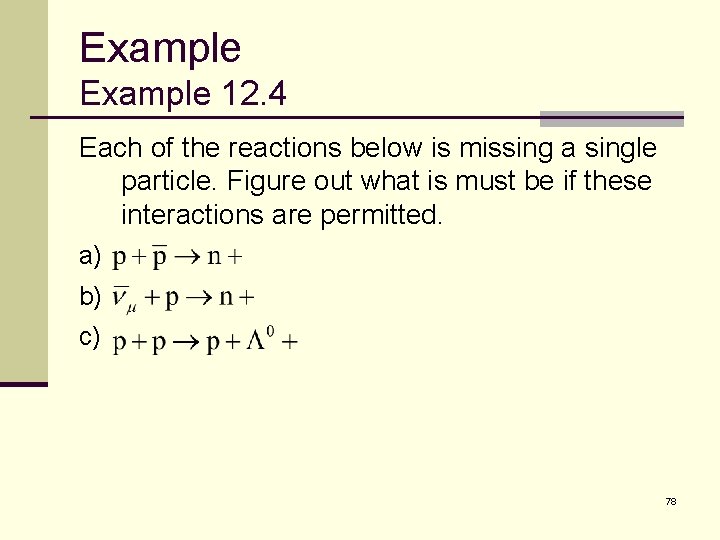
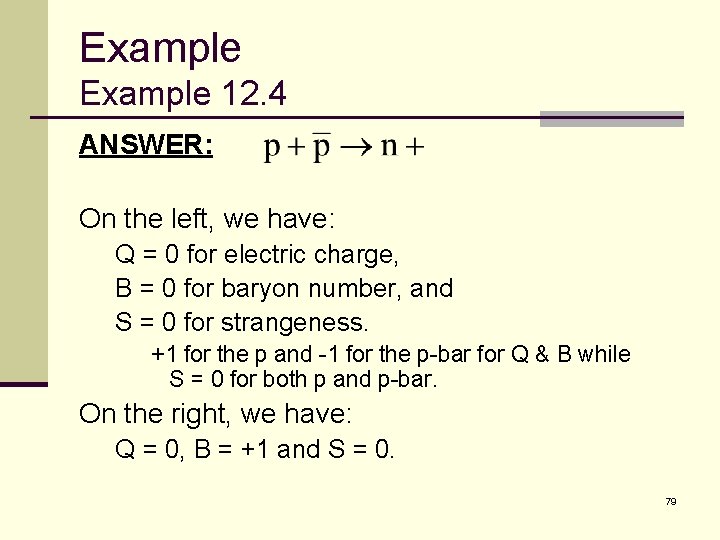
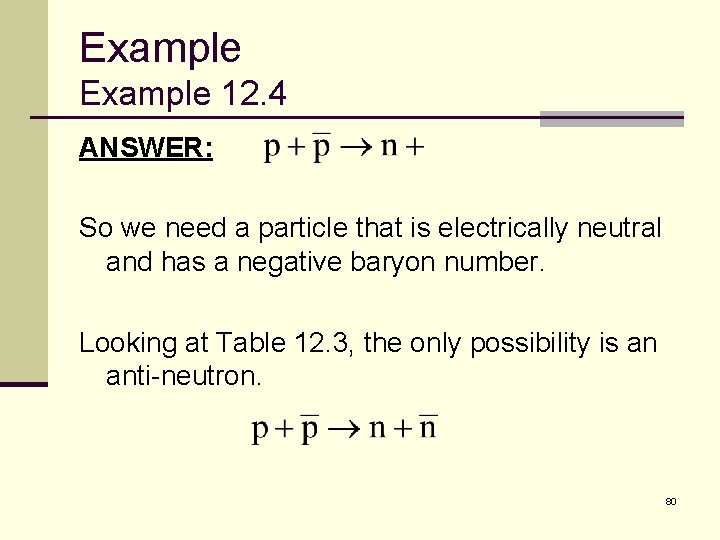
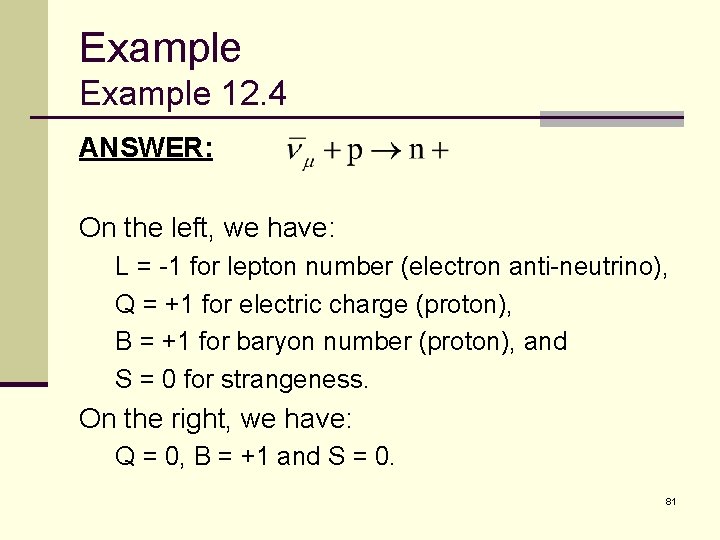
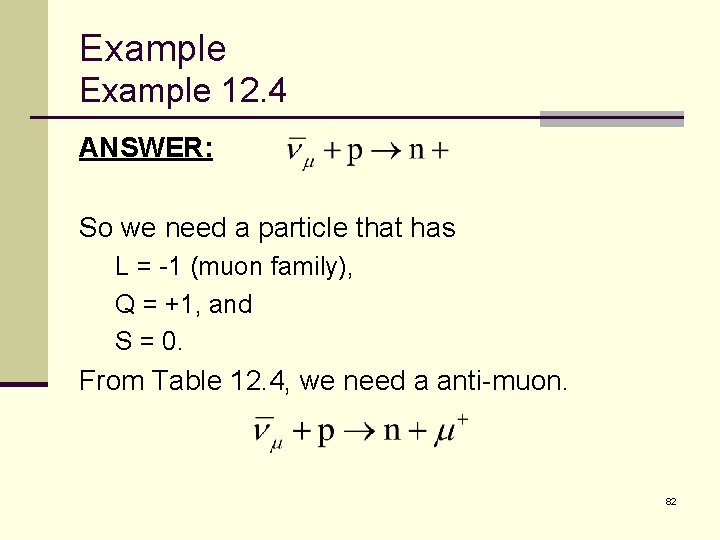
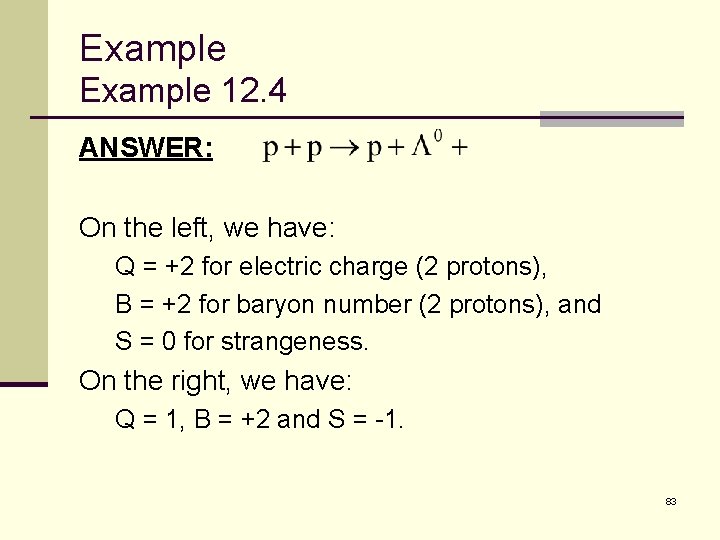
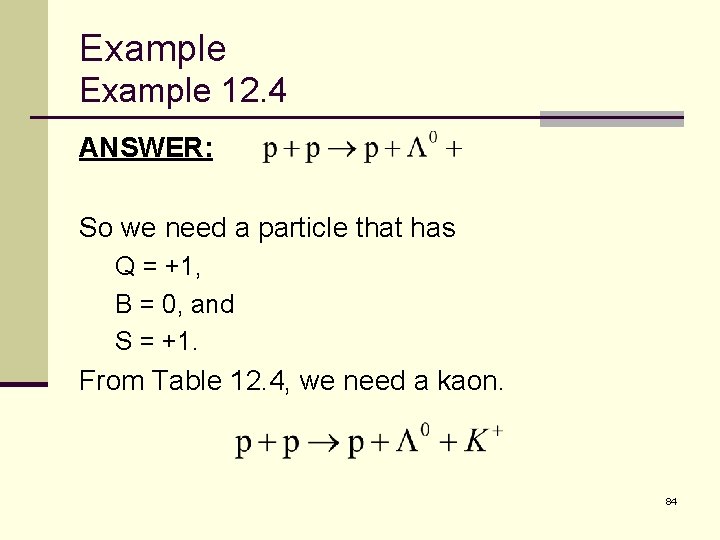
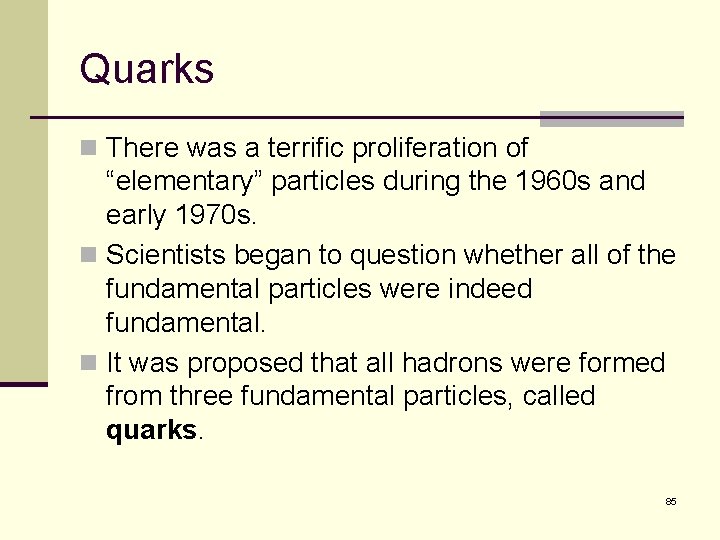
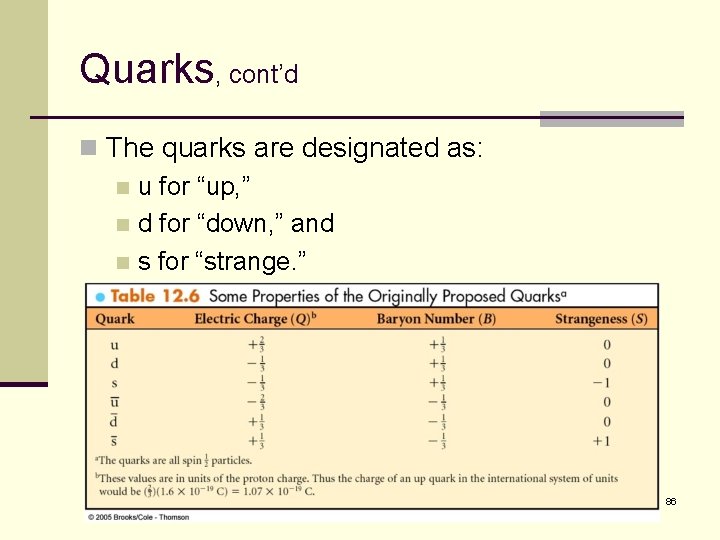
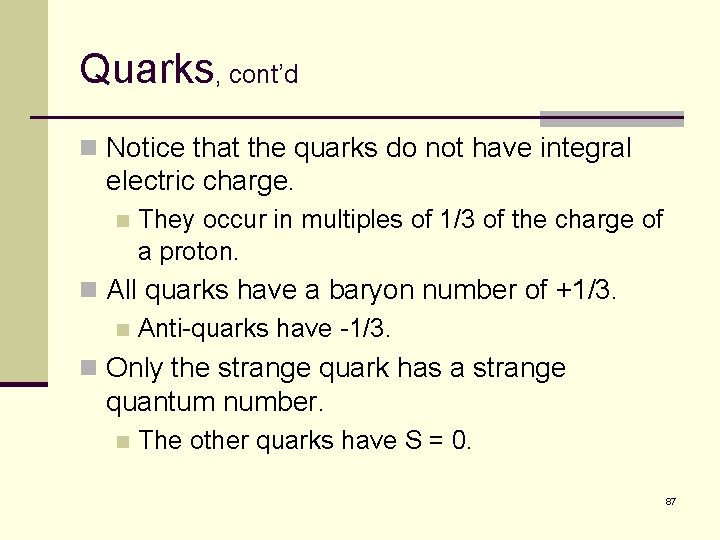
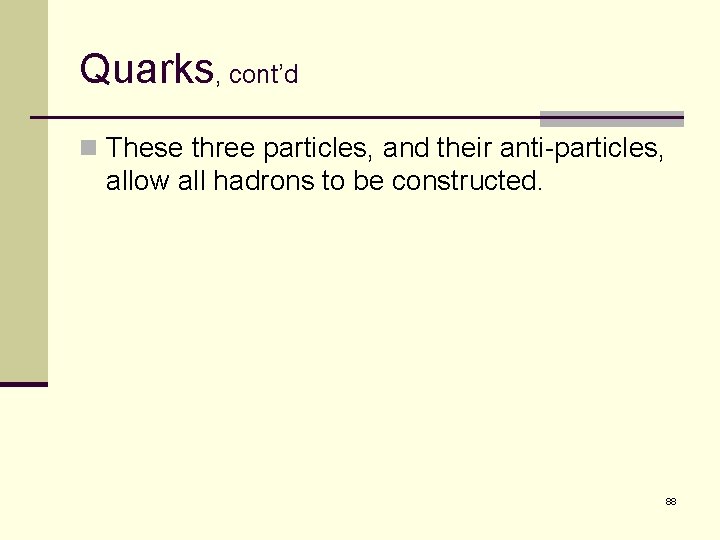
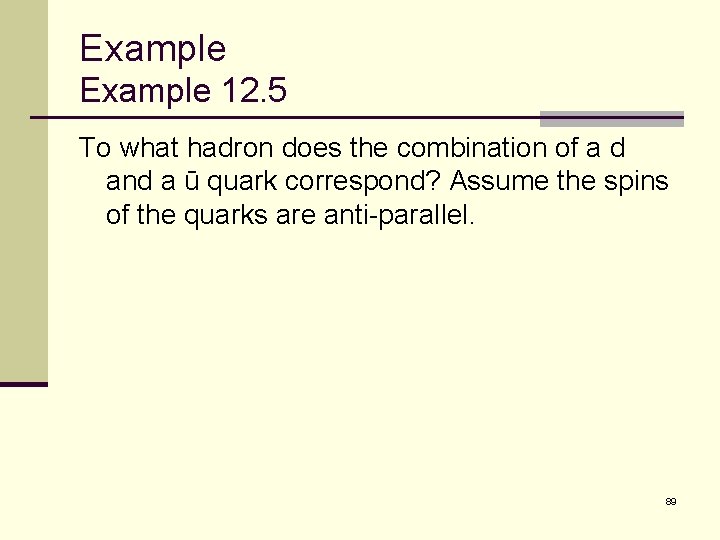
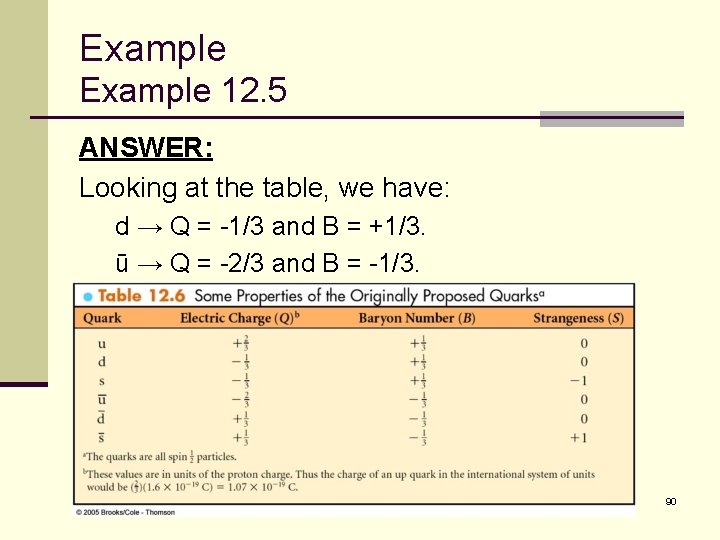
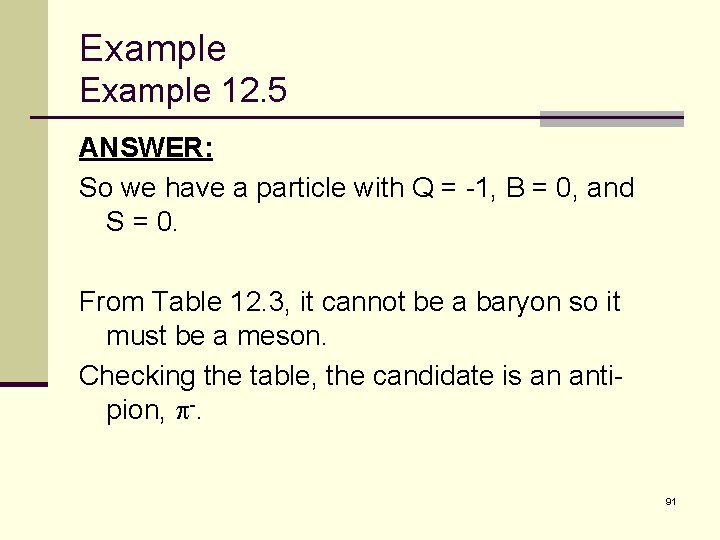
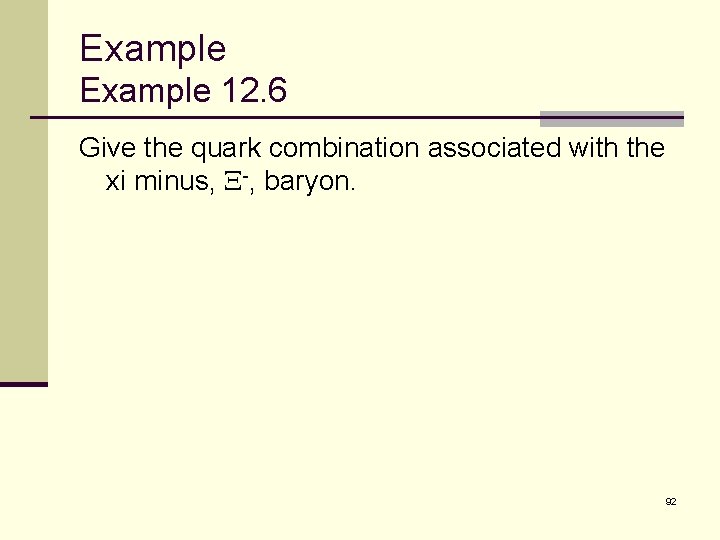
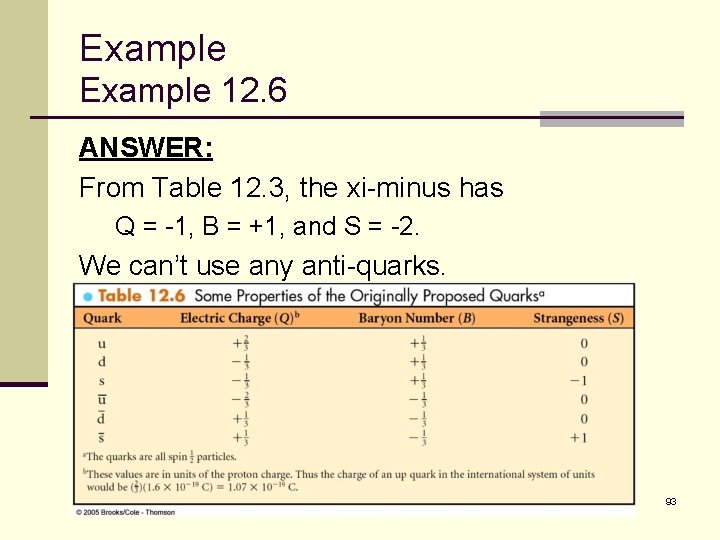
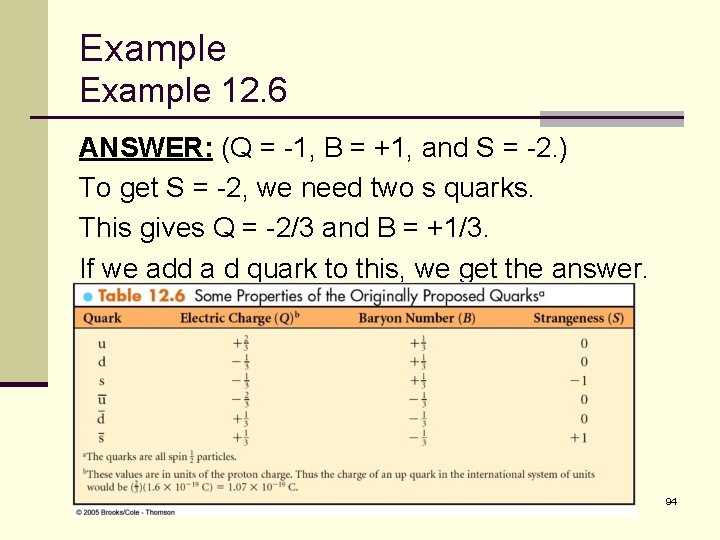
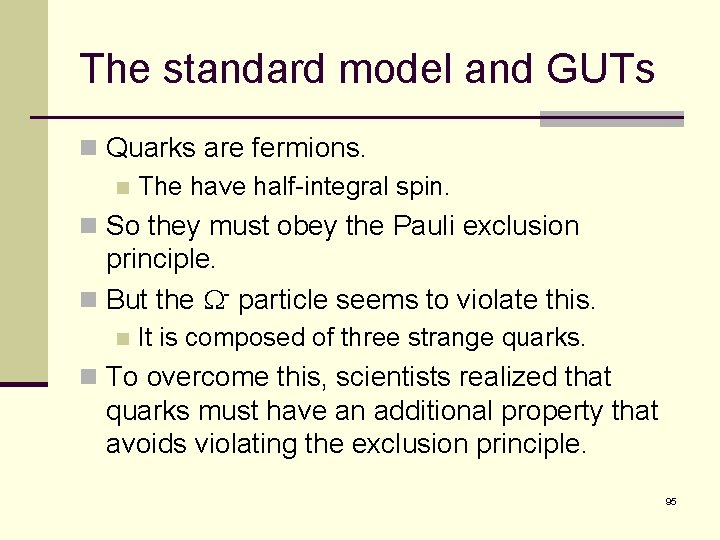
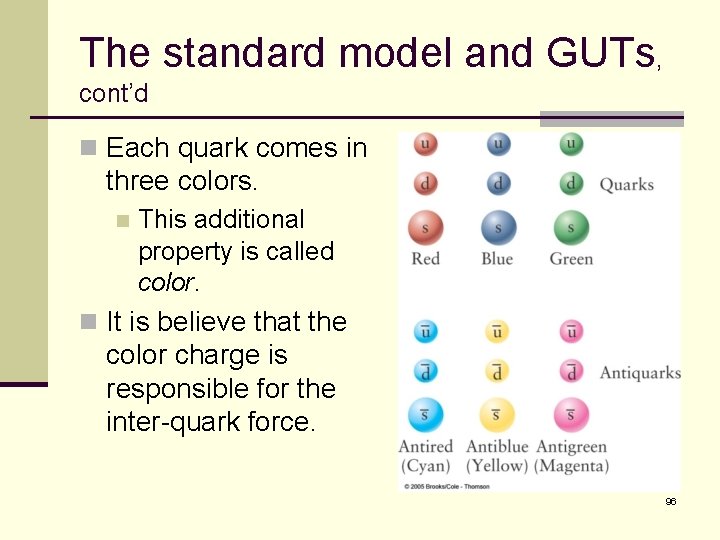
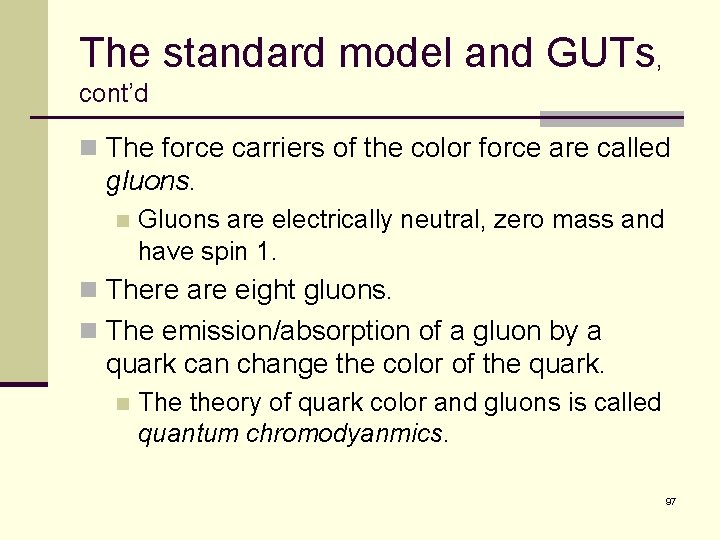
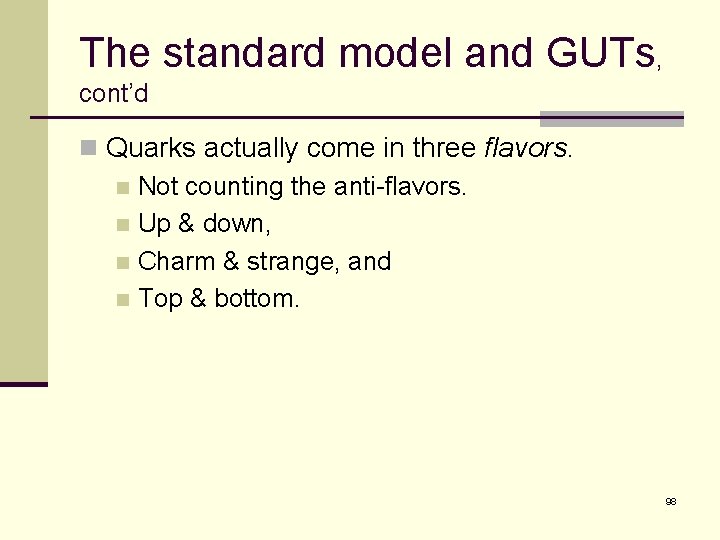
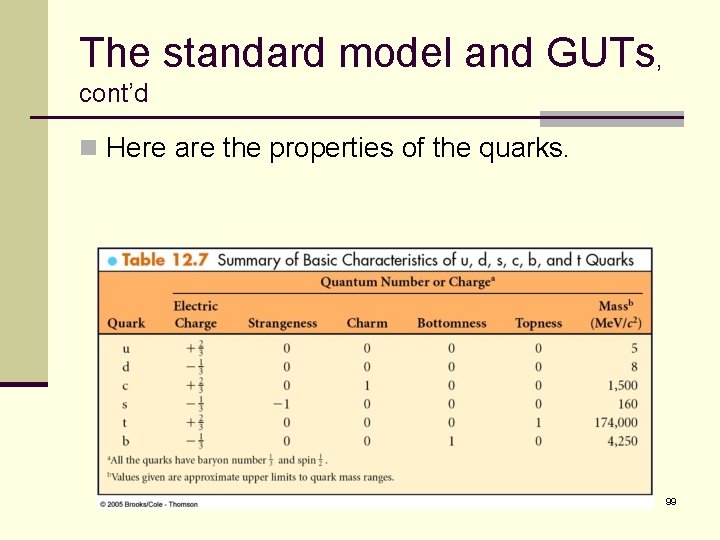
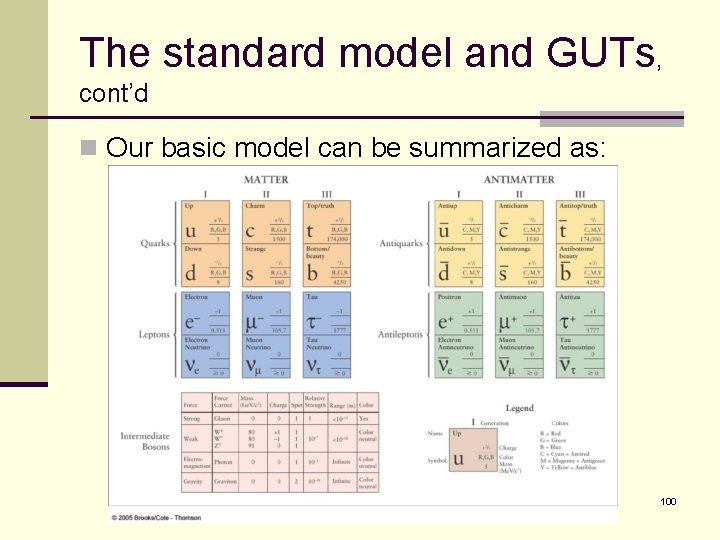
- Slides: 100
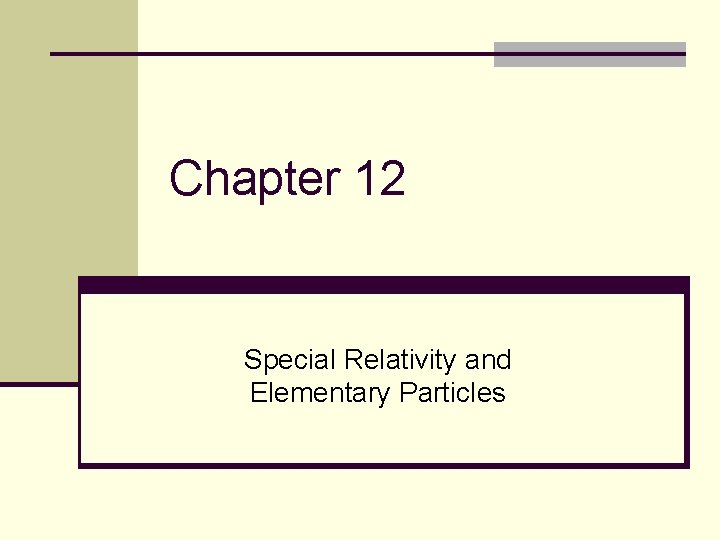
Chapter 12 Special Relativity and Elementary Particles
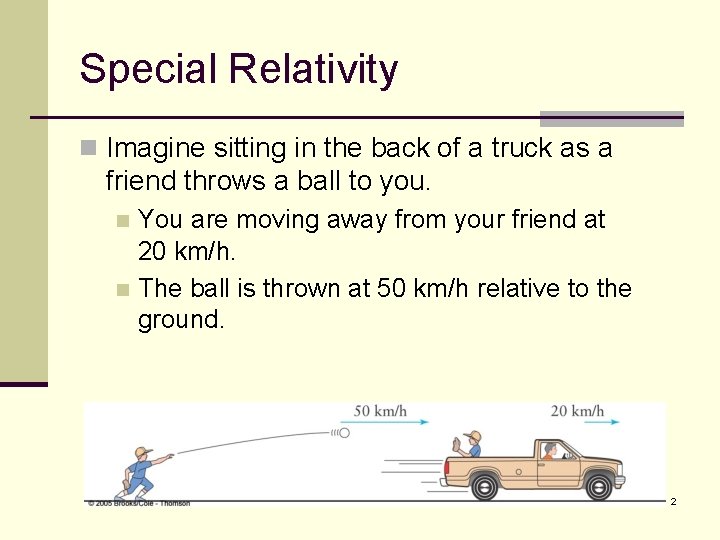
Special Relativity n Imagine sitting in the back of a truck as a friend throws a ball to you. You are moving away from your friend at 20 km/h. n The ball is thrown at 50 km/h relative to the ground. n 2
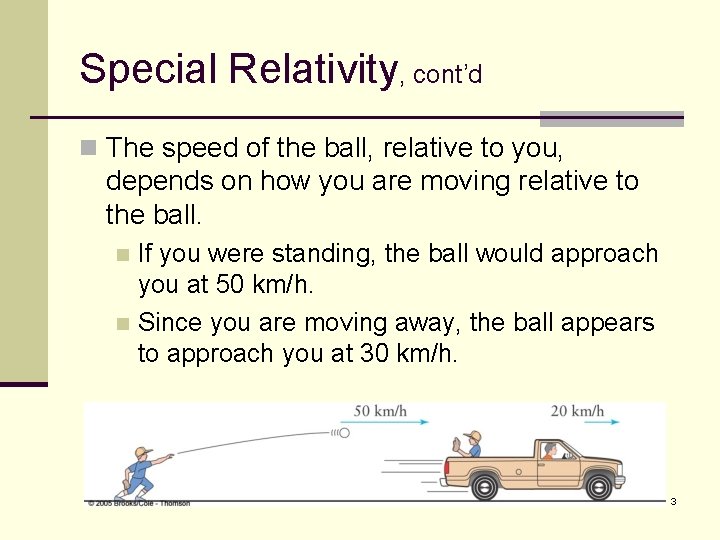
Special Relativity, cont’d n The speed of the ball, relative to you, depends on how you are moving relative to the ball. If you were standing, the ball would approach you at 50 km/h. n Since you are moving away, the ball appears to approach you at 30 km/h. n 3
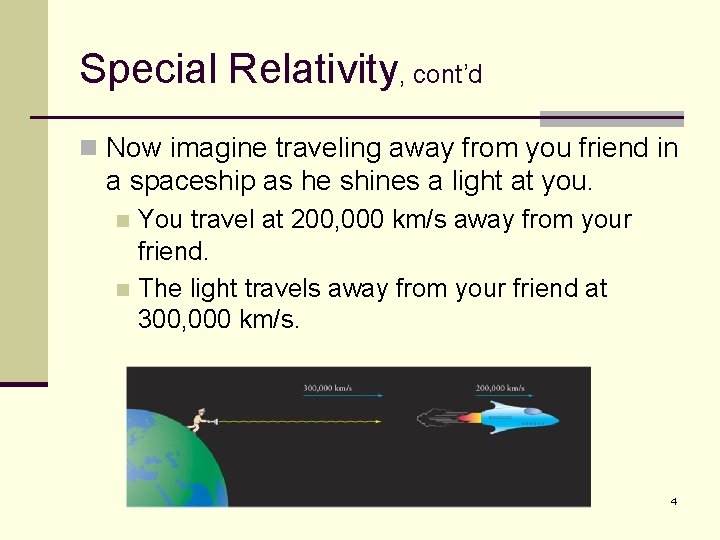
Special Relativity, cont’d n Now imagine traveling away from you friend in a spaceship as he shines a light at you. You travel at 200, 000 km/s away from your friend. n The light travels away from your friend at 300, 000 km/s. n 4
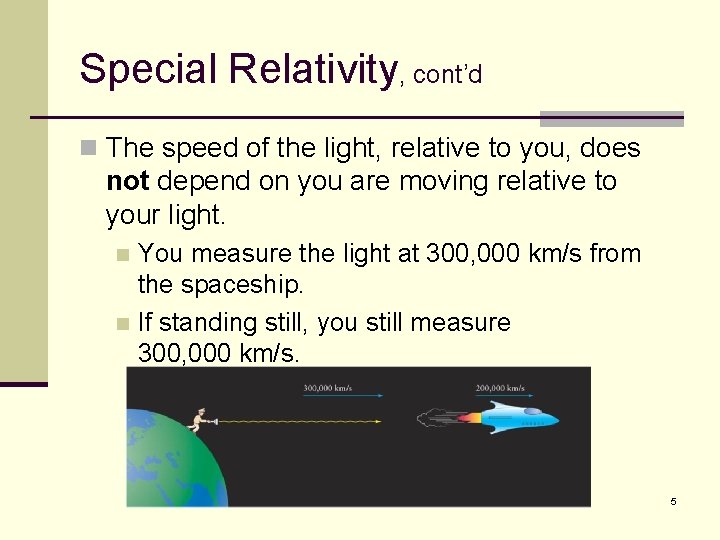
Special Relativity, cont’d n The speed of the light, relative to you, does not depend on you are moving relative to your light. You measure the light at 300, 000 km/s from the spaceship. n If standing still, you still measure 300, 000 km/s. n 5
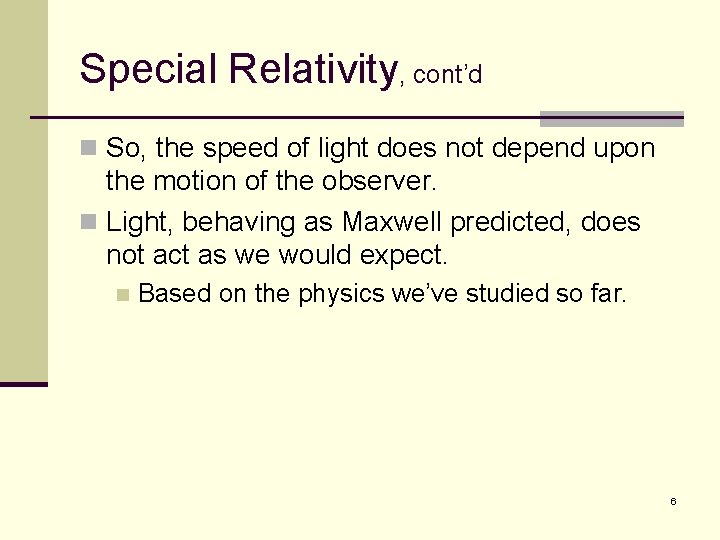
Special Relativity, cont’d n So, the speed of light does not depend upon the motion of the observer. n Light, behaving as Maxwell predicted, does not act as we would expect. n Based on the physics we’ve studied so far. 6
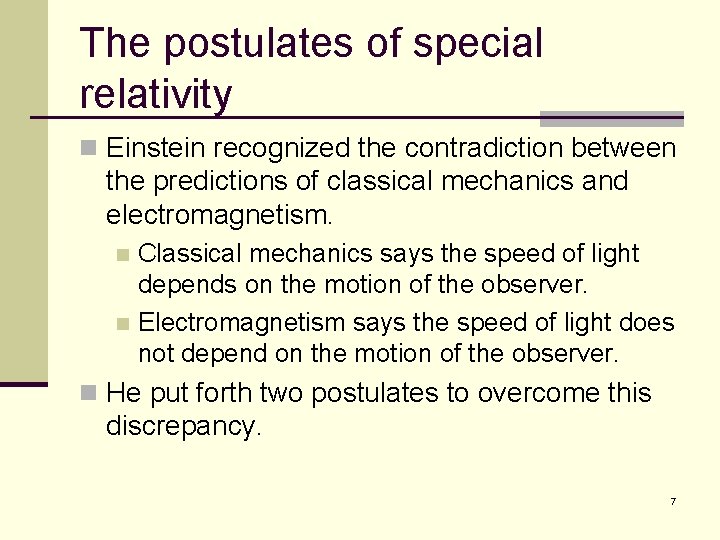
The postulates of special relativity n Einstein recognized the contradiction between the predictions of classical mechanics and electromagnetism. Classical mechanics says the speed of light depends on the motion of the observer. n Electromagnetism says the speed of light does not depend on the motion of the observer. n n He put forth two postulates to overcome this discrepancy. 7
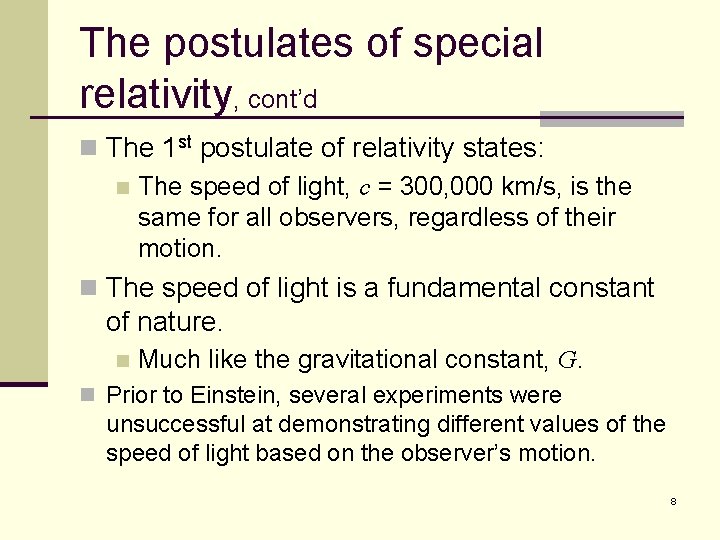
The postulates of special relativity, cont’d n The 1 st postulate of relativity states: n The speed of light, c = 300, 000 km/s, is the same for all observers, regardless of their motion. n The speed of light is a fundamental constant of nature. n Much like the gravitational constant, G. n Prior to Einstein, several experiments were unsuccessful at demonstrating different values of the speed of light based on the observer’s motion. 8
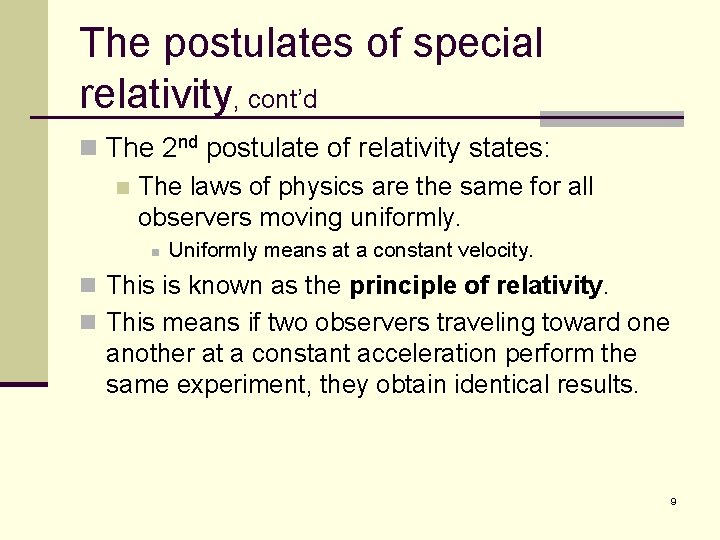
The postulates of special relativity, cont’d n The 2 nd postulate of relativity states: n The laws of physics are the same for all observers moving uniformly. n Uniformly means at a constant velocity. n This is known as the principle of relativity. n This means if two observers traveling toward one another at a constant acceleration perform the same experiment, they obtain identical results. 9
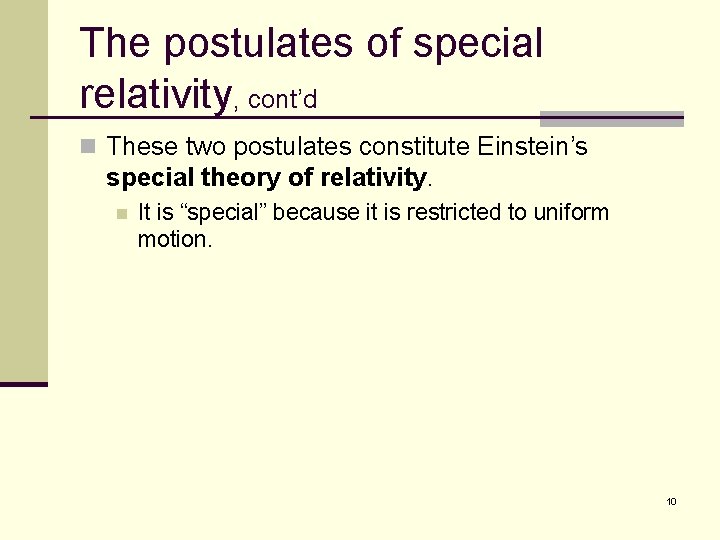
The postulates of special relativity, cont’d n These two postulates constitute Einstein’s special theory of relativity. n It is “special” because it is restricted to uniform motion. 10
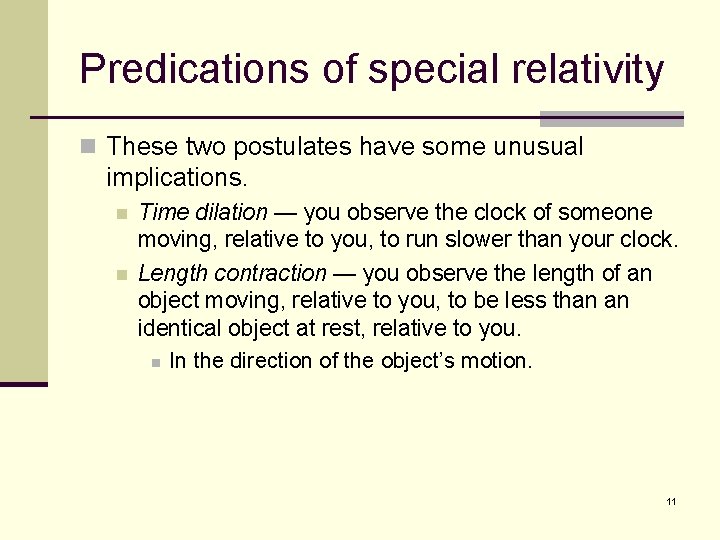
Predications of special relativity n These two postulates have some unusual implications. n n Time dilation — you observe the clock of someone moving, relative to you, to run slower than your clock. Length contraction — you observe the length of an object moving, relative to you, to be less than an identical object at rest, relative to you. n In the direction of the object’s motion. 11
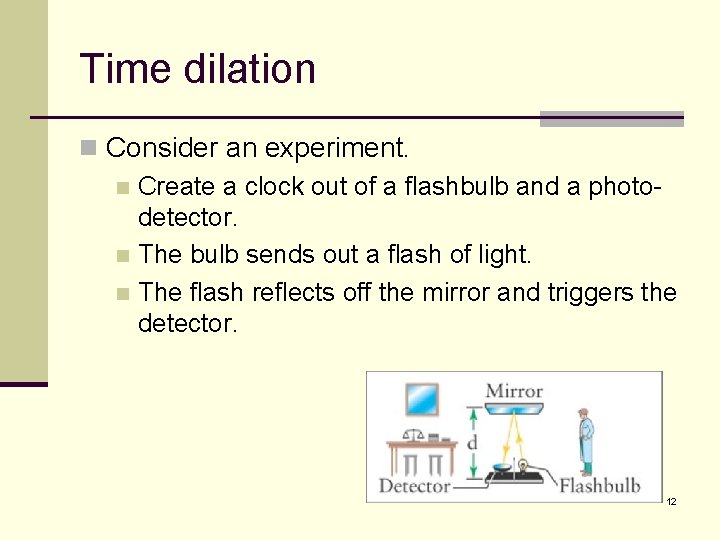
Time dilation n Consider an experiment. n Create a clock out of a flashbulb and a photodetector. n The bulb sends out a flash of light. n The flash reflects off the mirror and triggers the detector. 12
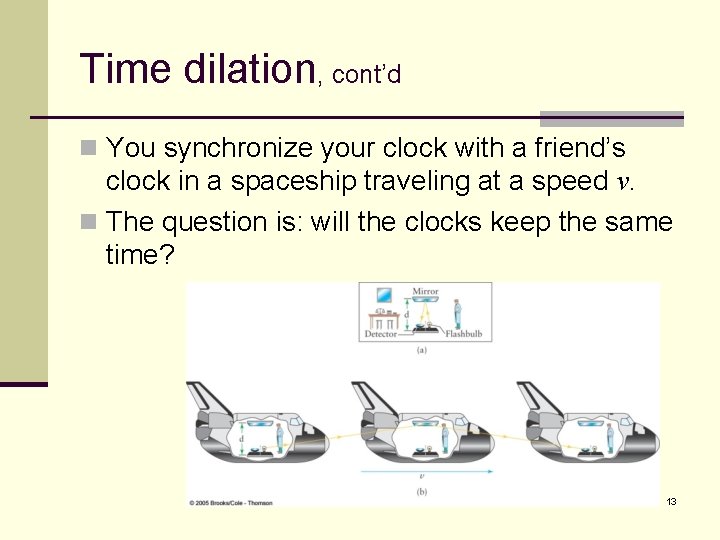
Time dilation, cont’d n You synchronize your clock with a friend’s clock in a spaceship traveling at a speed v. n The question is: will the clocks keep the same time? 13
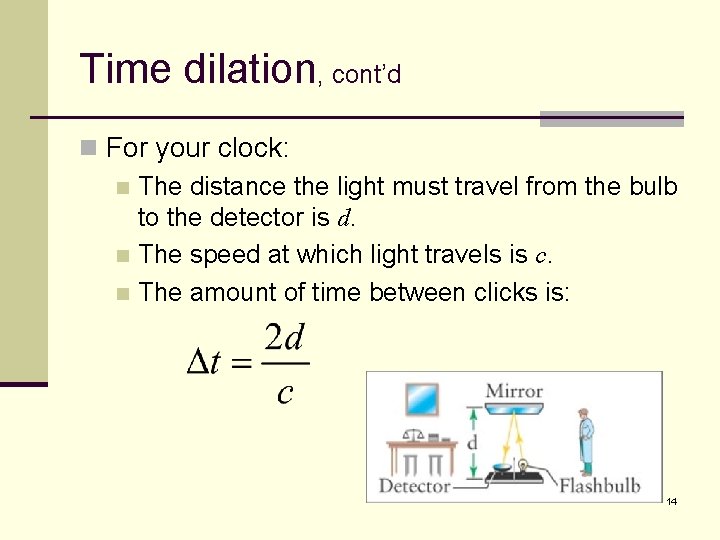
Time dilation, cont’d n For your clock: n The distance the light must travel from the bulb to the detector is d. n The speed at which light travels is c. n The amount of time between clicks is: 14
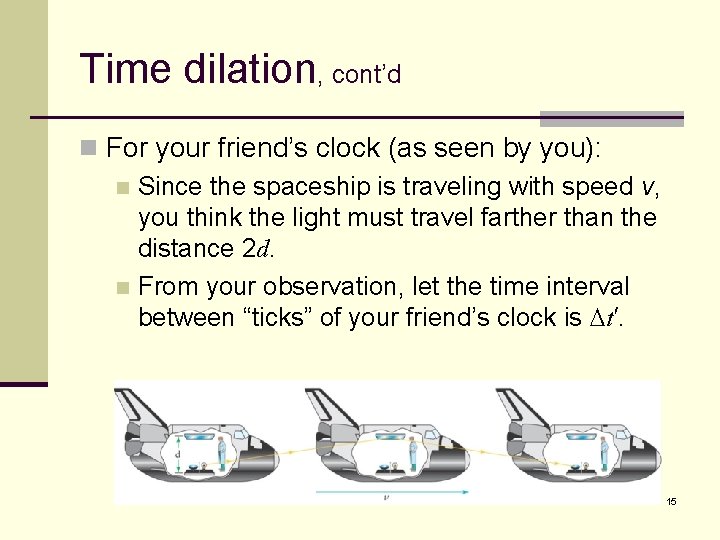
Time dilation, cont’d n For your friend’s clock (as seen by you): n Since the spaceship is traveling with speed v, you think the light must travel farther than the distance 2 d. n From your observation, let the time interval between “ticks” of your friend’s clock is Dt′. 15
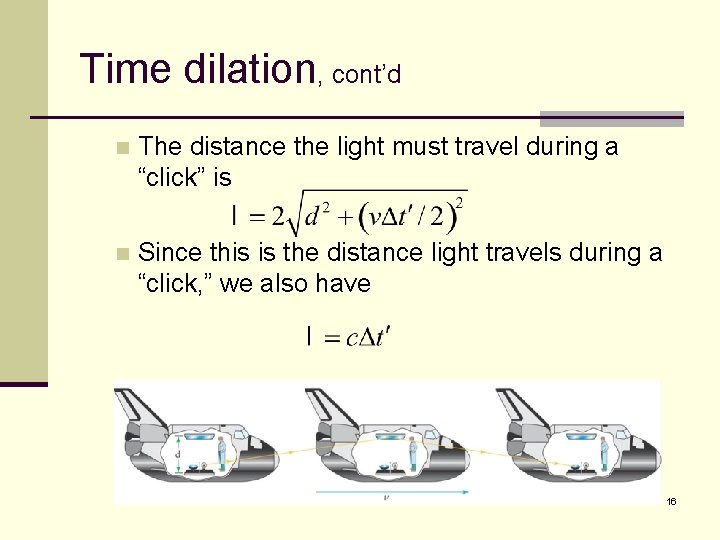
Time dilation, cont’d n The distance the light must travel during a “click” is n Since this is the distance light travels during a “click, ” we also have 16
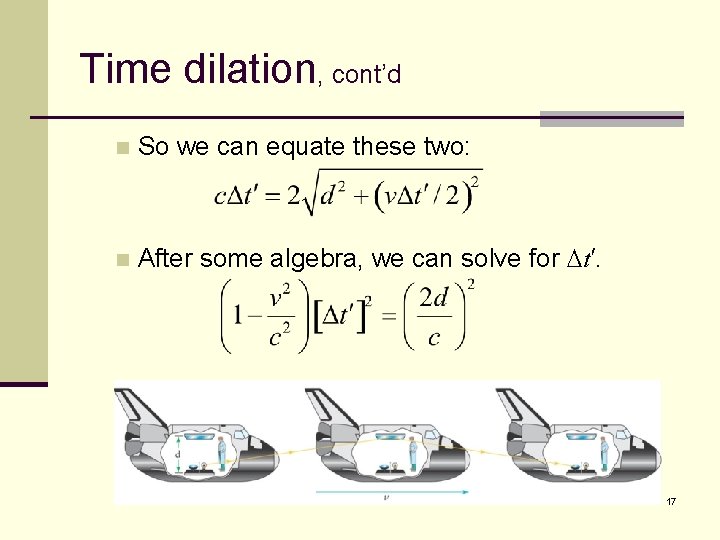
Time dilation, cont’d n So we can equate these two: n After some algebra, we can solve for Dt′. 17
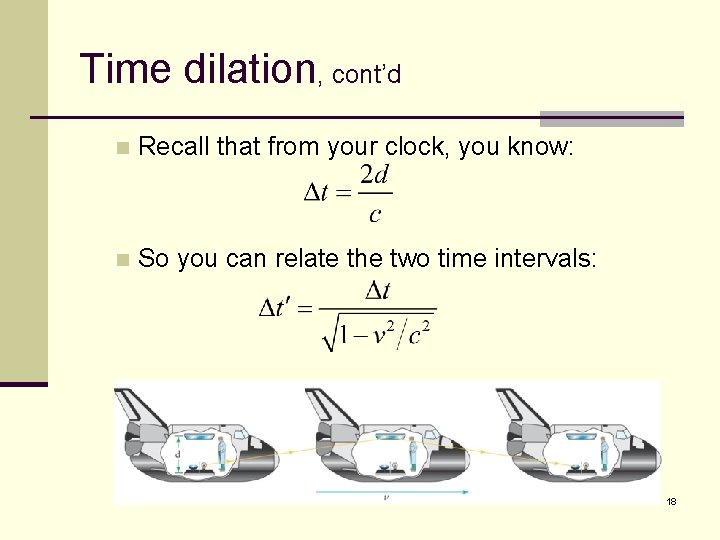
Time dilation, cont’d n Recall that from your clock, you know: n So you can relate the two time intervals: 18
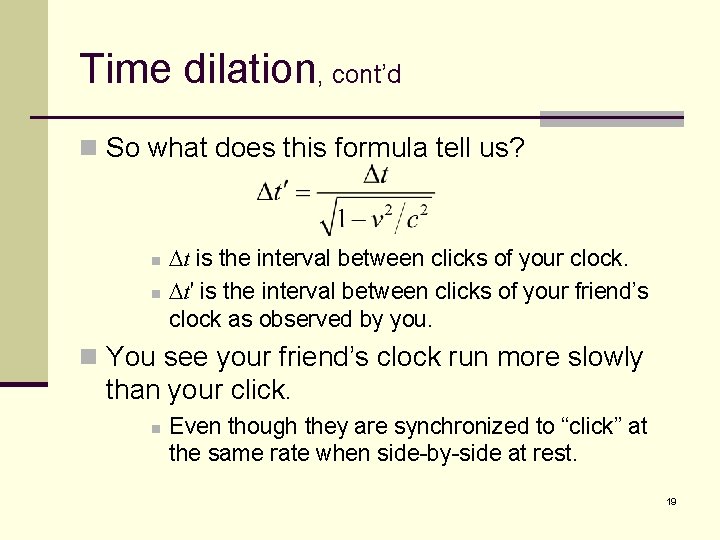
Time dilation, cont’d n So what does this formula tell us? n n Dt is the interval between clicks of your clock. Dt′ is the interval between clicks of your friend’s clock as observed by you. n You see your friend’s clock run more slowly than your click. n Even though they are synchronized to “click” at the same rate when side-by-side at rest. 19
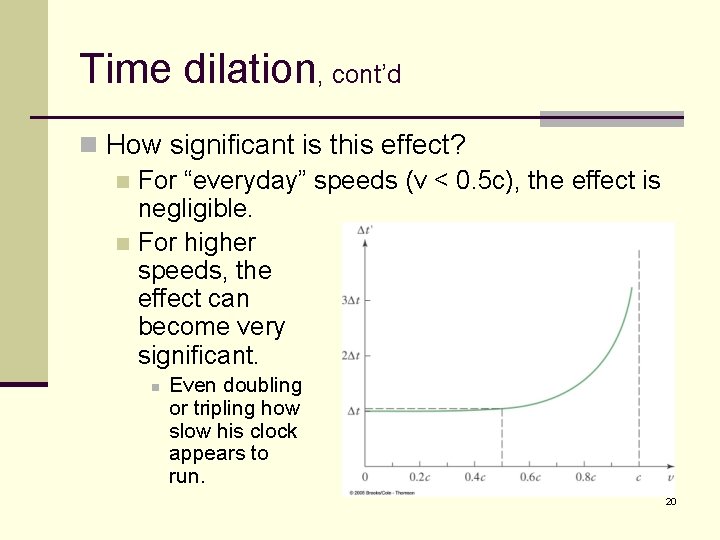
Time dilation, cont’d n How significant is this effect? n For “everyday” speeds (v < 0. 5 c), the effect is negligible. n For higher speeds, the effect can become very significant. n Even doubling or tripling how slow his clock appears to run. 20
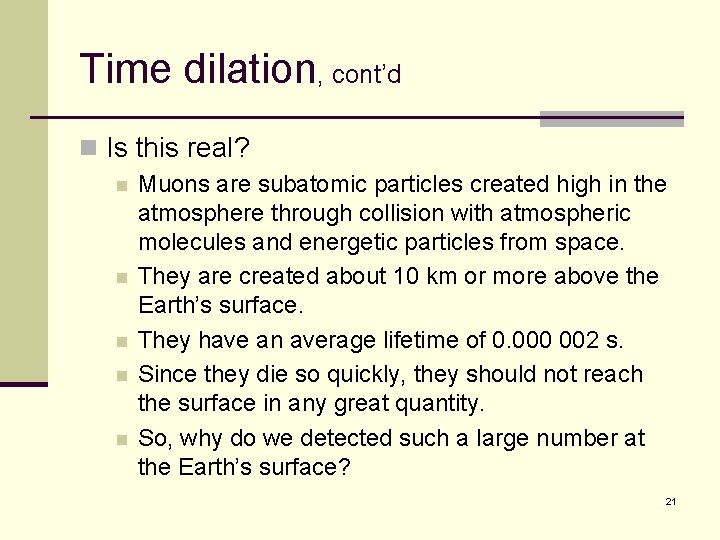
Time dilation, cont’d n Is this real? n n n Muons are subatomic particles created high in the atmosphere through collision with atmospheric molecules and energetic particles from space. They are created about 10 km or more above the Earth’s surface. They have an average lifetime of 0. 000 002 s. Since they die so quickly, they should not reach the surface in any great quantity. So, why do we detected such a large number at the Earth’s surface? 21
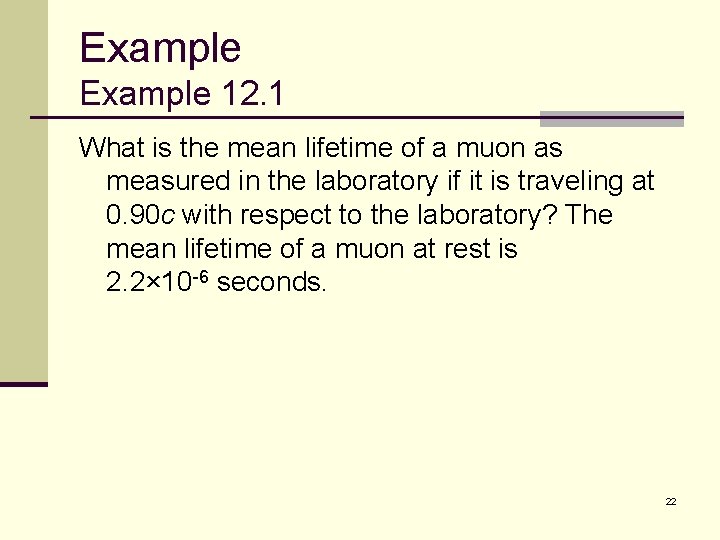
Example 12. 1 What is the mean lifetime of a muon as measured in the laboratory if it is traveling at 0. 90 c with respect to the laboratory? The mean lifetime of a muon at rest is 2. 2× 10 -6 seconds. 22
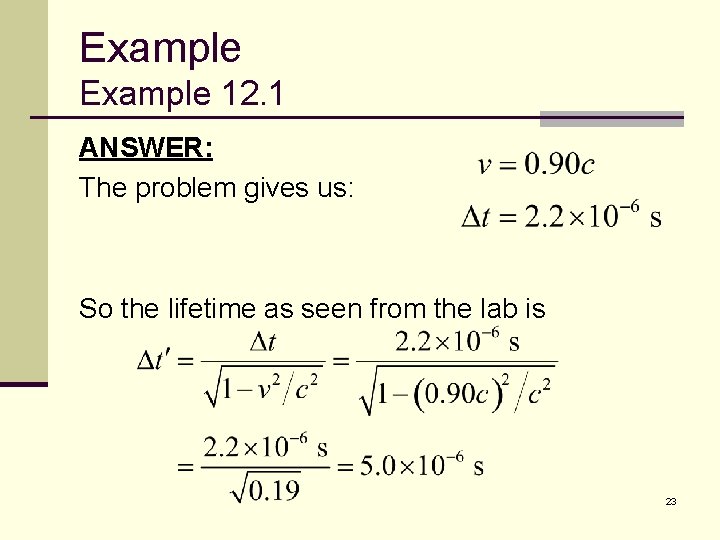
Example 12. 1 ANSWER: The problem gives us: So the lifetime as seen from the lab is 23
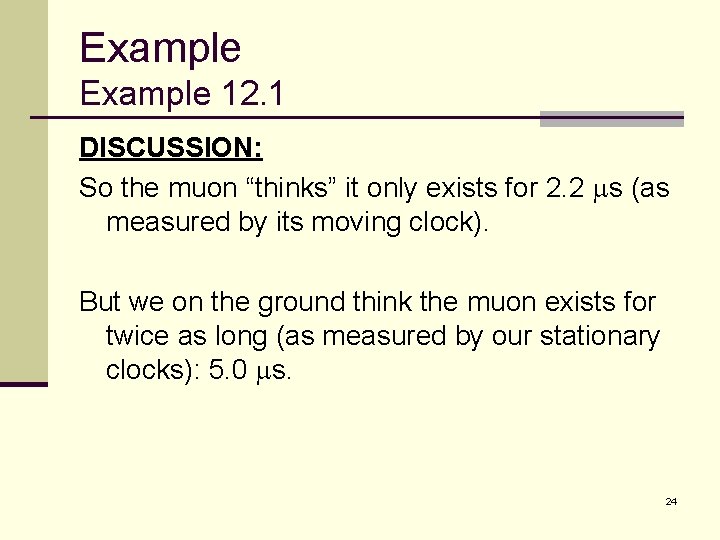
Example 12. 1 DISCUSSION: So the muon “thinks” it only exists for 2. 2 ms (as measured by its moving clock). But we on the ground think the muon exists for twice as long (as measured by our stationary clocks): 5. 0 ms. 24
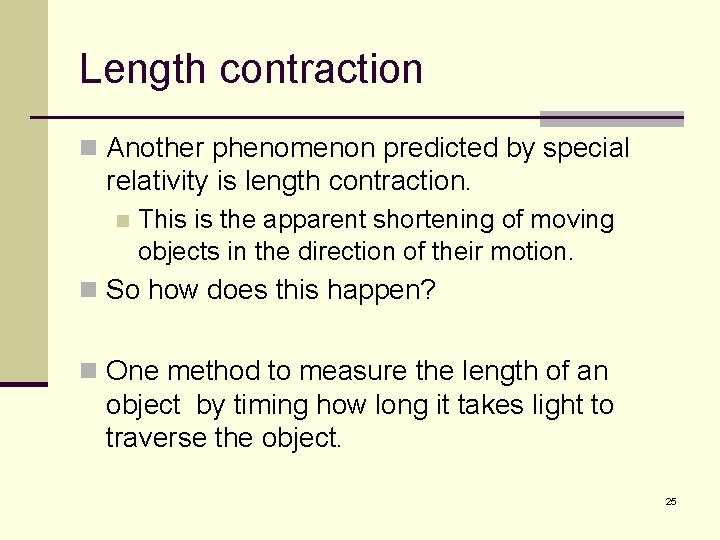
Length contraction n Another phenomenon predicted by special relativity is length contraction. n This is the apparent shortening of moving objects in the direction of their motion. n So how does this happen? n One method to measure the length of an object by timing how long it takes light to traverse the object. 25
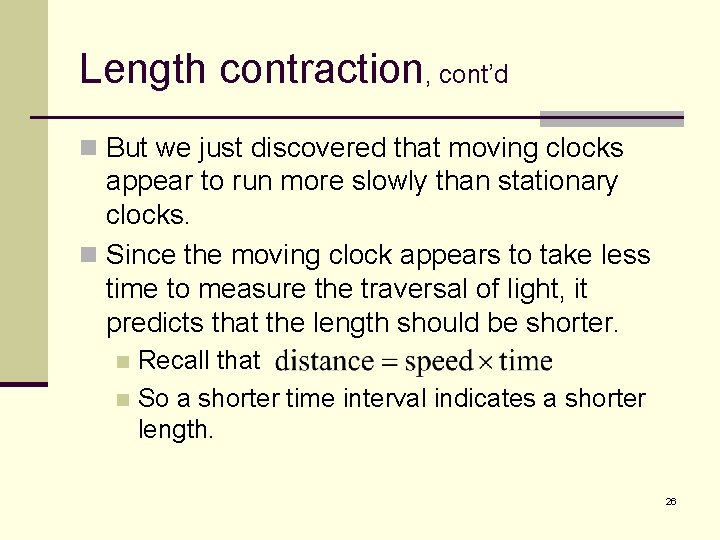
Length contraction, cont’d n But we just discovered that moving clocks appear to run more slowly than stationary clocks. n Since the moving clock appears to take less time to measure the traversal of light, it predicts that the length should be shorter. Recall that n So a shorter time interval indicates a shorter length. n 26
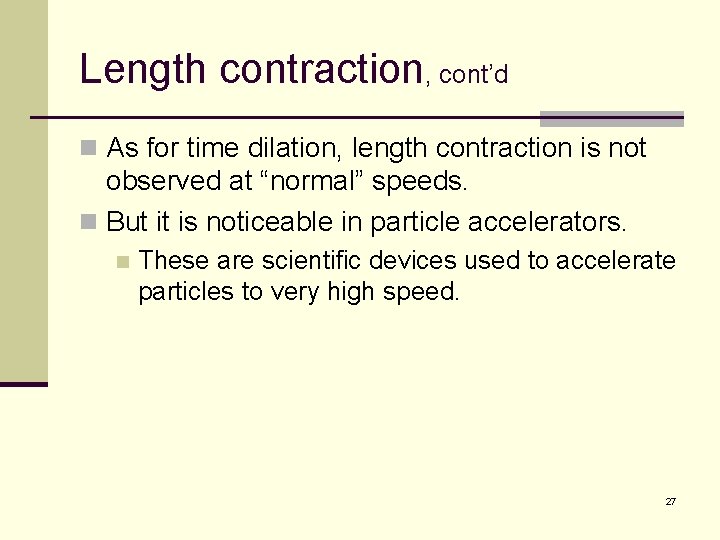
Length contraction, cont’d n As for time dilation, length contraction is not observed at “normal” speeds. n But it is noticeable in particle accelerators. n These are scientific devices used to accelerate particles to very high speed. 27
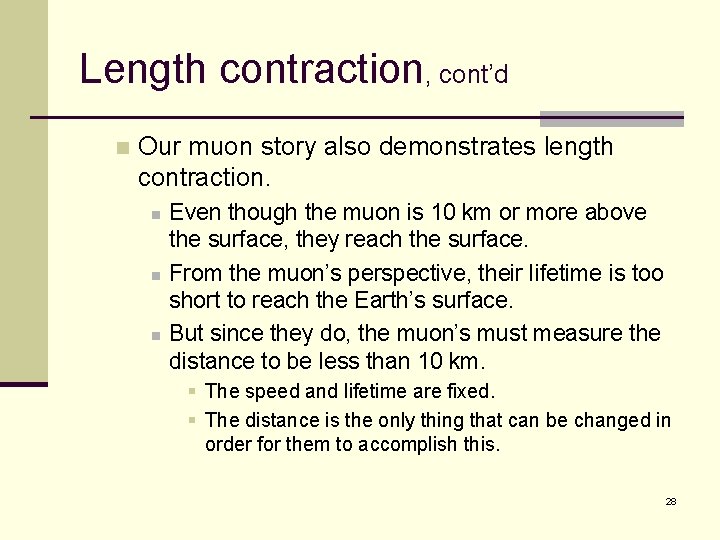
Length contraction, cont’d n Our muon story also demonstrates length contraction. n n n Even though the muon is 10 km or more above the surface, they reach the surface. From the muon’s perspective, their lifetime is too short to reach the Earth’s surface. But since they do, the muon’s must measure the distance to be less than 10 km. § The speed and lifetime are fixed. § The distance is the only thing that can be changed in order for them to accomplish this. 28
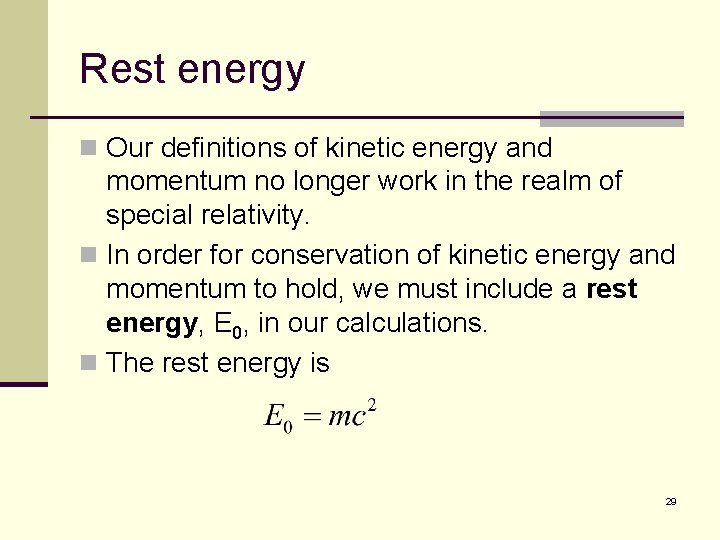
Rest energy n Our definitions of kinetic energy and momentum no longer work in the realm of special relativity. n In order for conservation of kinetic energy and momentum to hold, we must include a rest energy, E 0, in our calculations. n The rest energy is 29
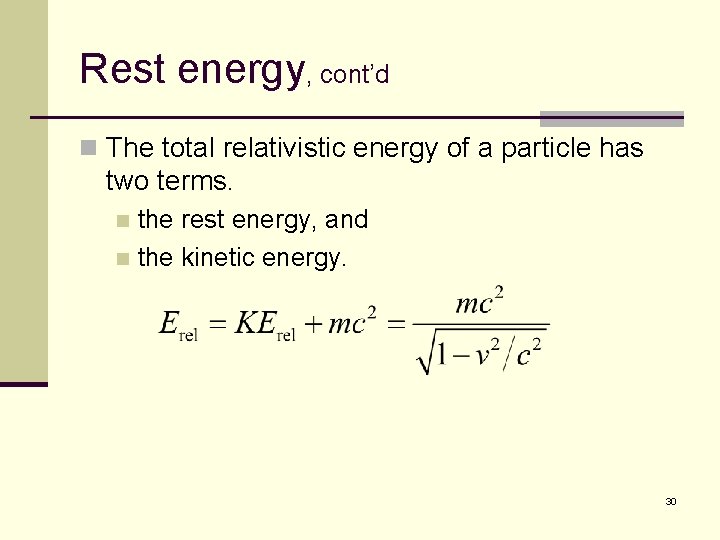
Rest energy, cont’d n The total relativistic energy of a particle has two terms. the rest energy, and n the kinetic energy. n 30
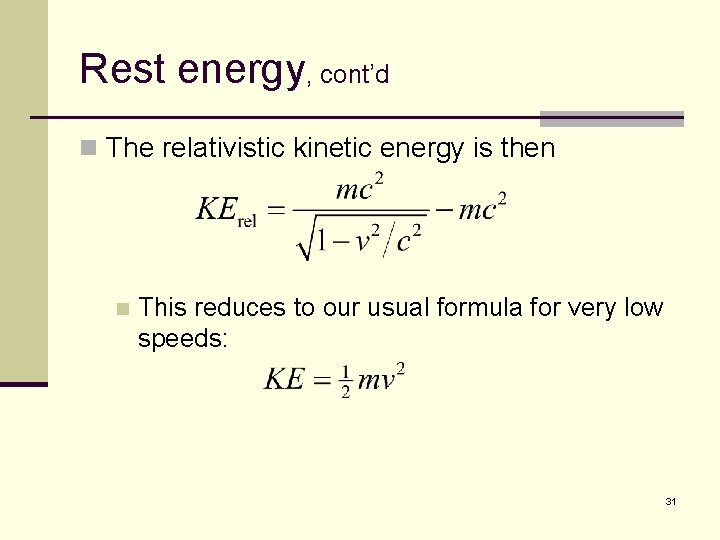
Rest energy, cont’d n The relativistic kinetic energy is then n This reduces to our usual formula for very low speeds: 31
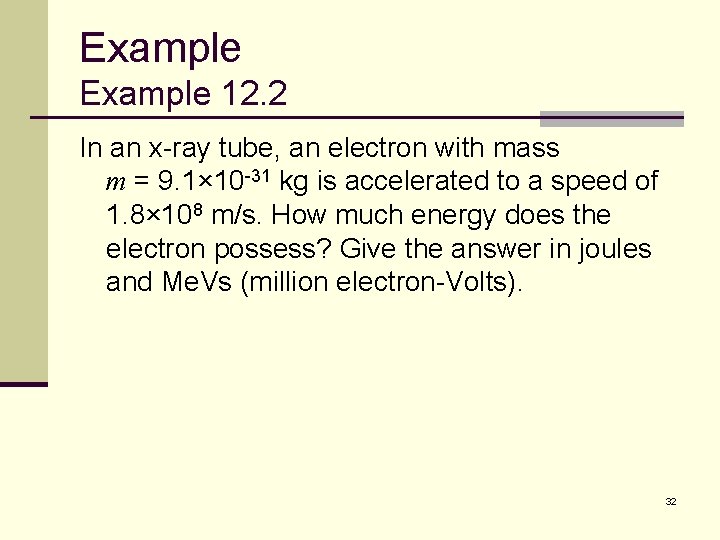
Example 12. 2 In an x-ray tube, an electron with mass m = 9. 1× 10 -31 kg is accelerated to a speed of 1. 8× 108 m/s. How much energy does the electron possess? Give the answer in joules and Me. Vs (million electron-Volts). 32
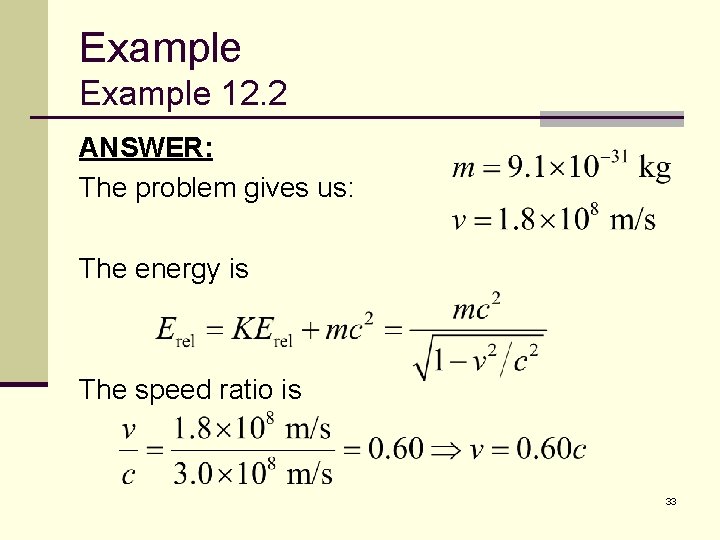
Example 12. 2 ANSWER: The problem gives us: The energy is The speed ratio is 33
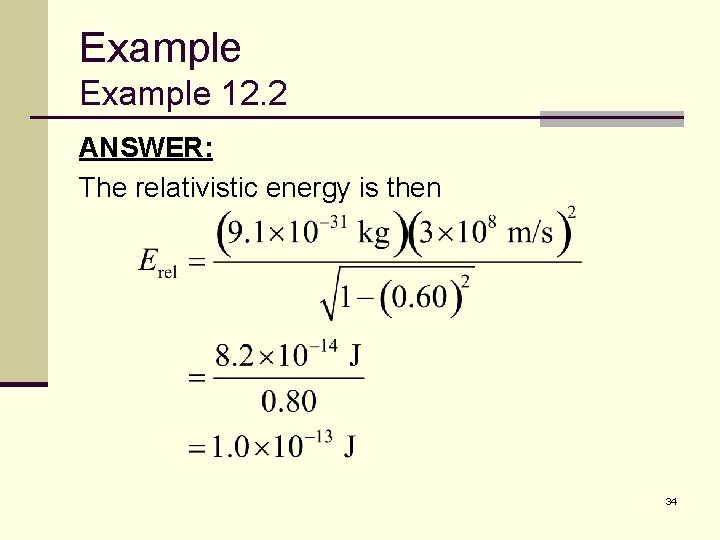
Example 12. 2 ANSWER: The relativistic energy is then 34
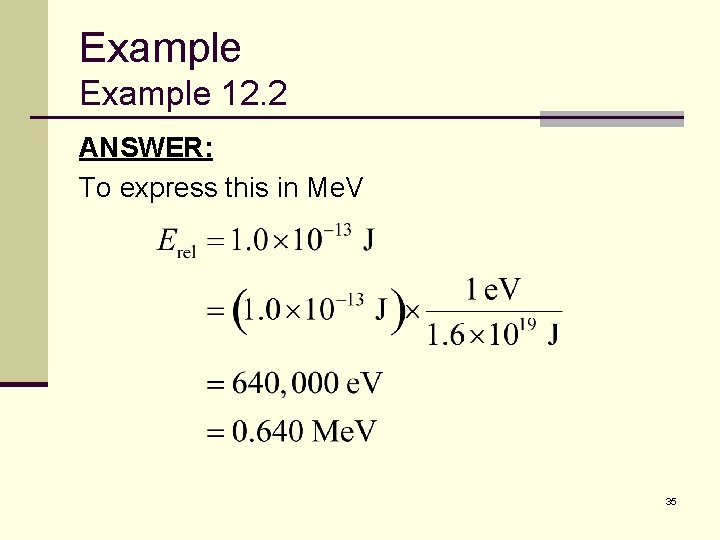
Example 12. 2 ANSWER: To express this in Me. V 35
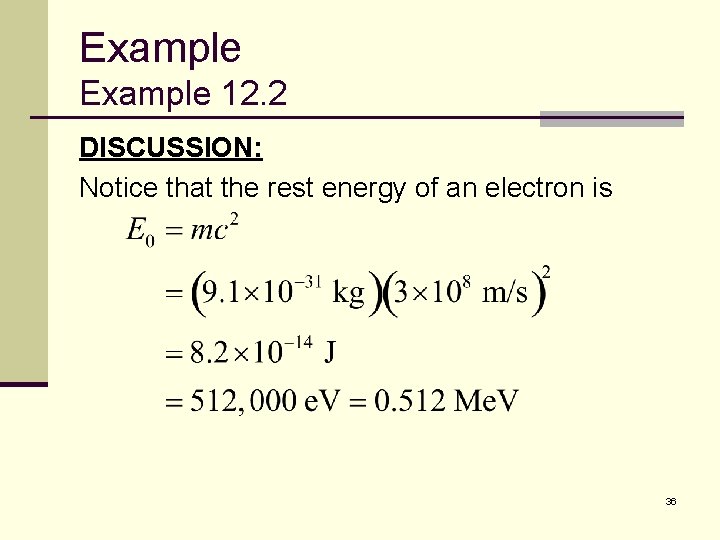
Example 12. 2 DISCUSSION: Notice that the rest energy of an electron is 36
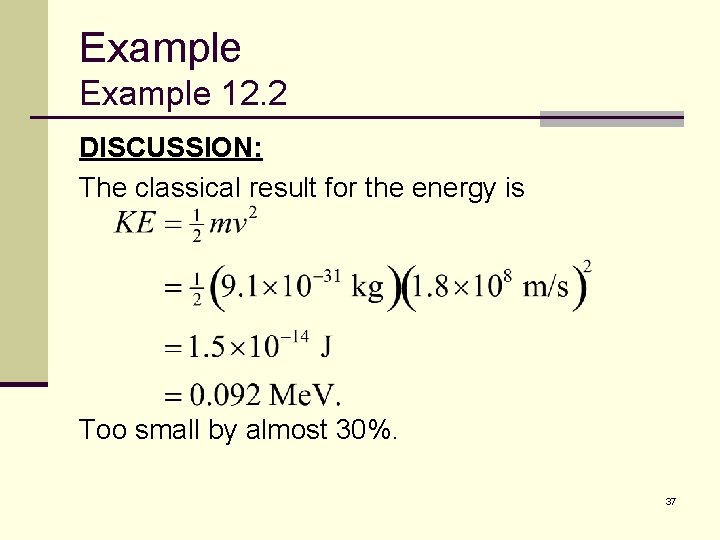
Example 12. 2 DISCUSSION: The classical result for the energy is Too small by almost 30%. 37
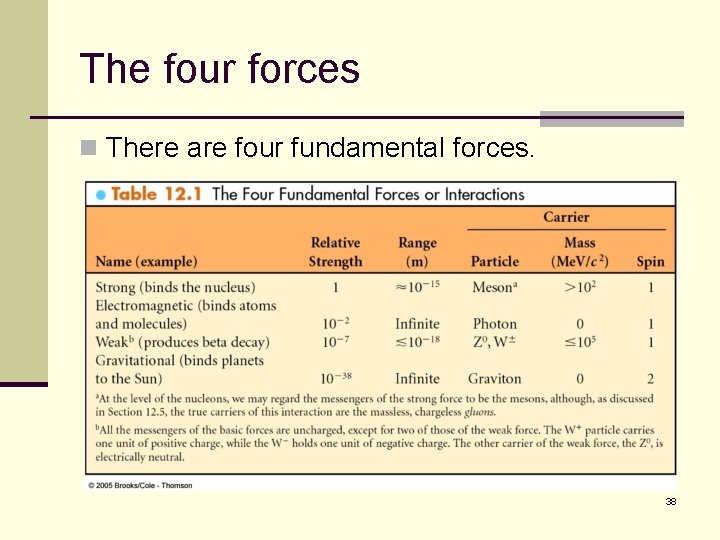
The four forces n There are four fundamental forces. 38
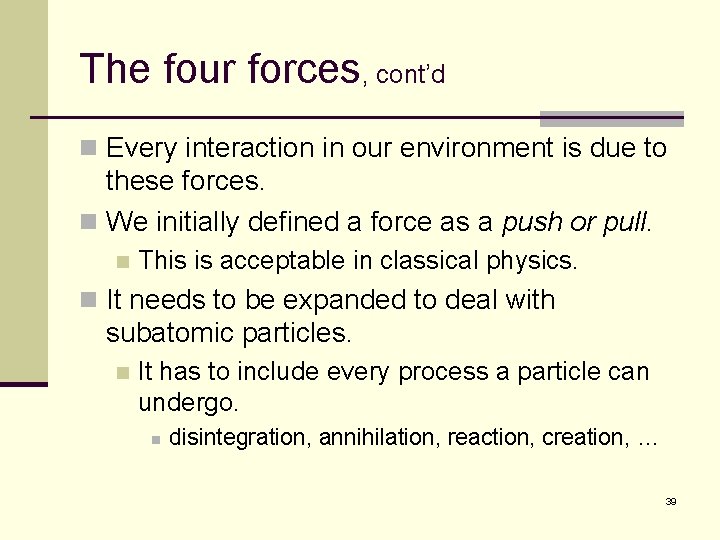
The four forces, cont’d n Every interaction in our environment is due to these forces. n We initially defined a force as a push or pull. n This is acceptable in classical physics. n It needs to be expanded to deal with subatomic particles. n It has to include every process a particle can undergo. n disintegration, annihilation, reaction, creation, … 39
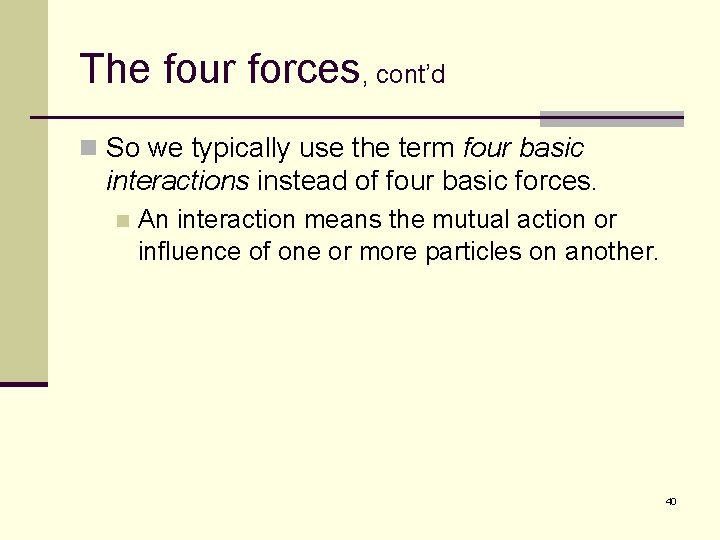
The four forces, cont’d n So we typically use the term four basic interactions instead of four basic forces. n An interaction means the mutual action or influence of one or more particles on another. 40
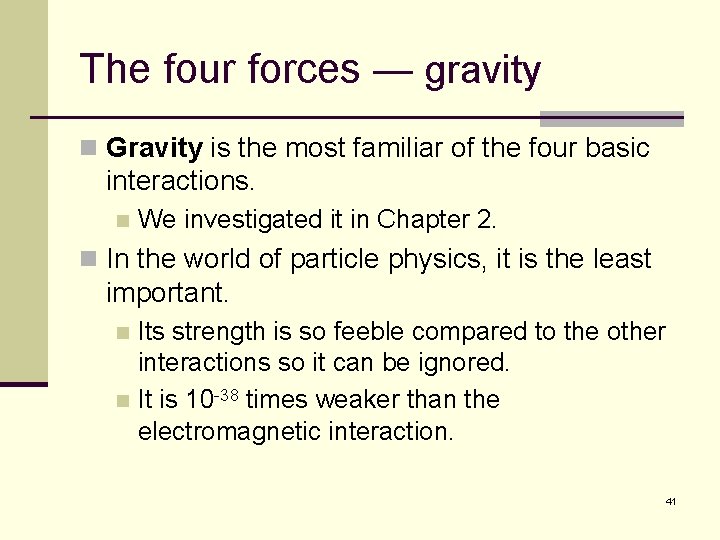
The four forces — gravity n Gravity is the most familiar of the four basic interactions. n We investigated it in Chapter 2. n In the world of particle physics, it is the least important. Its strength is so feeble compared to the other interactions so it can be ignored. n It is 10 -38 times weaker than the electromagnetic interaction. n 41
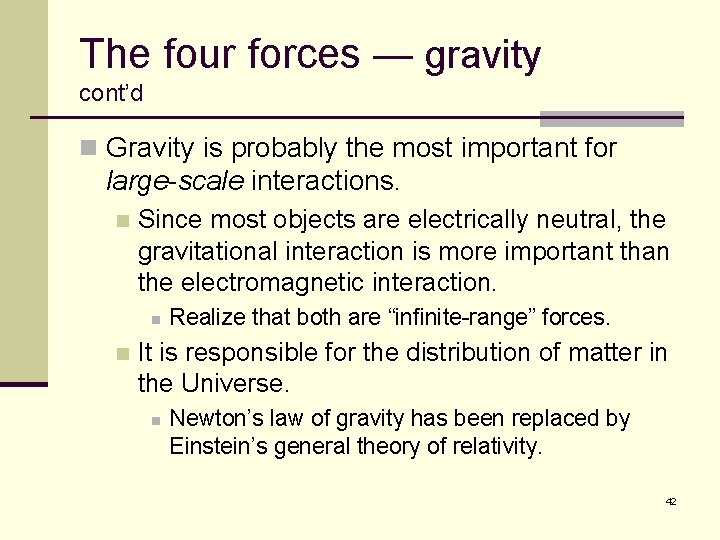
The four forces — gravity cont’d n Gravity is probably the most important for large-scale interactions. n Since most objects are electrically neutral, the gravitational interaction is more important than the electromagnetic interaction. n n Realize that both are “infinite-range” forces. It is responsible for the distribution of matter in the Universe. n Newton’s law of gravity has been replaced by Einstein’s general theory of relativity. 42
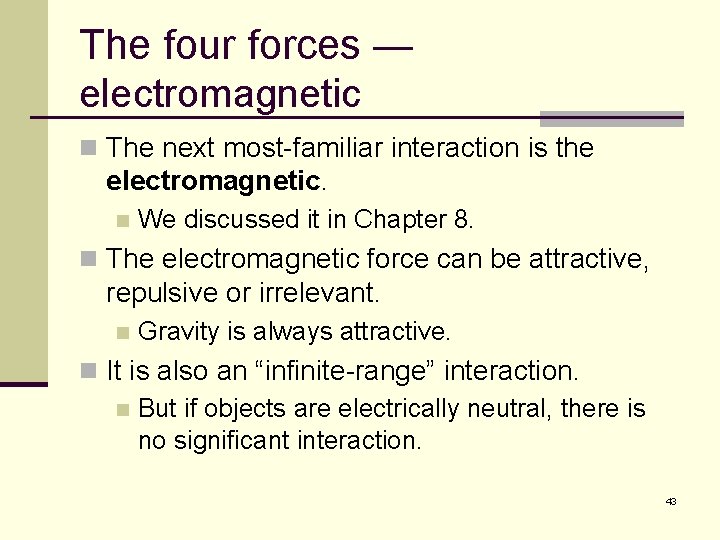
The four forces — electromagnetic n The next most-familiar interaction is the electromagnetic. n We discussed it in Chapter 8. n The electromagnetic force can be attractive, repulsive or irrelevant. n Gravity is always attractive. n It is also an “infinite-range” interaction. n But if objects are electrically neutral, there is no significant interaction. 43
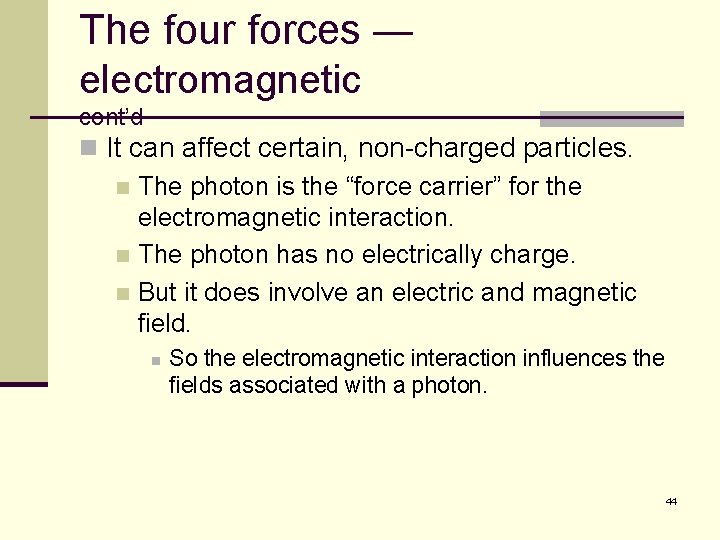
The four forces — electromagnetic cont’d n It can affect certain, non-charged particles. n The photon is the “force carrier” for the electromagnetic interaction. n The photon has no electrically charge. n But it does involve an electric and magnetic field. n So the electromagnetic interaction influences the fields associated with a photon. 44
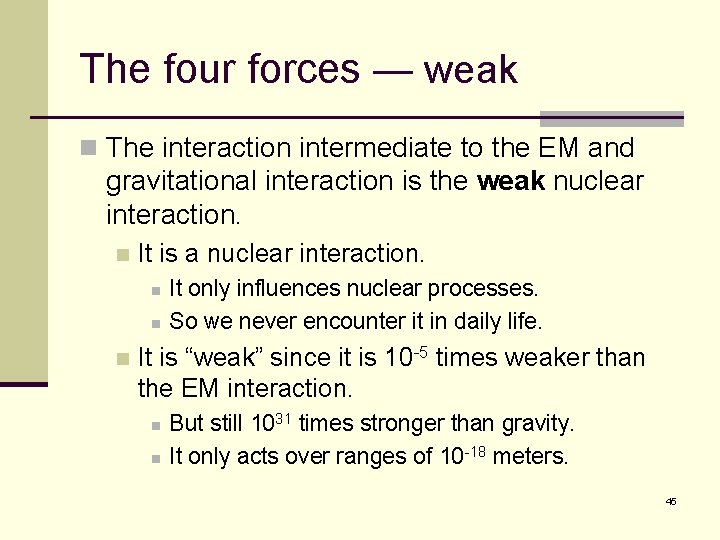
The four forces — weak n The interaction intermediate to the EM and gravitational interaction is the weak nuclear interaction. n It is a nuclear interaction. n n n It only influences nuclear processes. So we never encounter it in daily life. It is “weak” since it is 10 -5 times weaker than the EM interaction. n n But still 1031 times stronger than gravity. It only acts over ranges of 10 -18 meters. 45
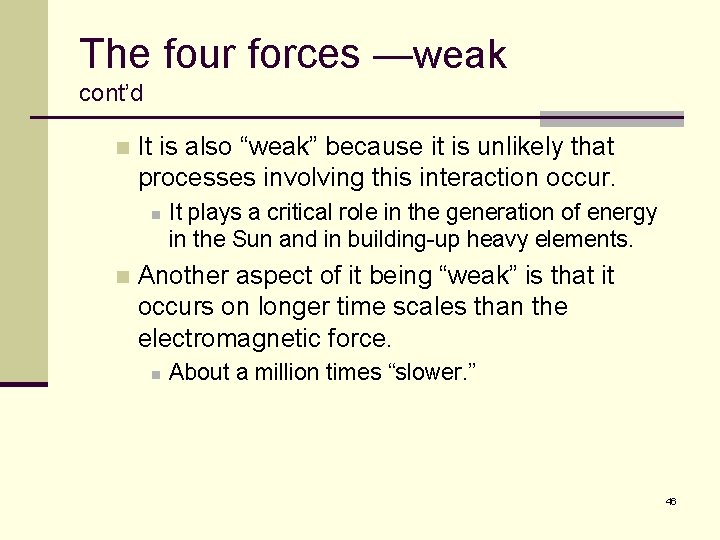
The four forces —weak cont’d n It is also “weak” because it is unlikely that processes involving this interaction occur. n n It plays a critical role in the generation of energy in the Sun and in building-up heavy elements. Another aspect of it being “weak” is that it occurs on longer time scales than the electromagnetic force. n About a million times “slower. ” 46
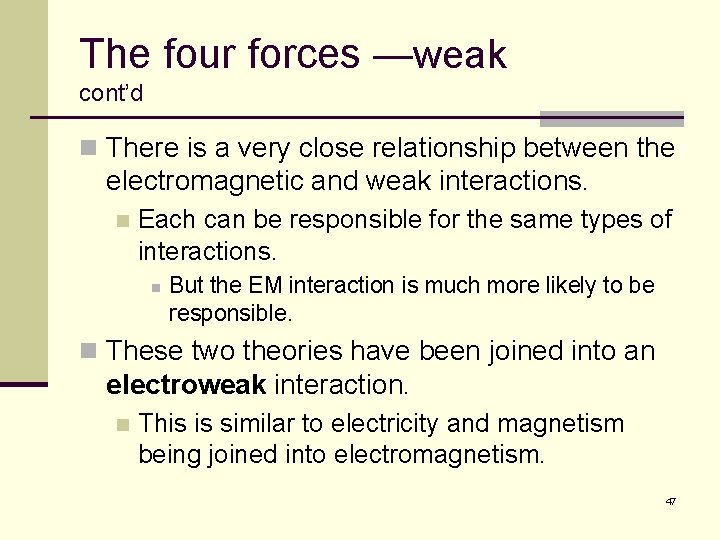
The four forces —weak cont’d n There is a very close relationship between the electromagnetic and weak interactions. n Each can be responsible for the same types of interactions. n But the EM interaction is much more likely to be responsible. n These two theories have been joined into an electroweak interaction. n This is similar to electricity and magnetism being joined into electromagnetism. 47
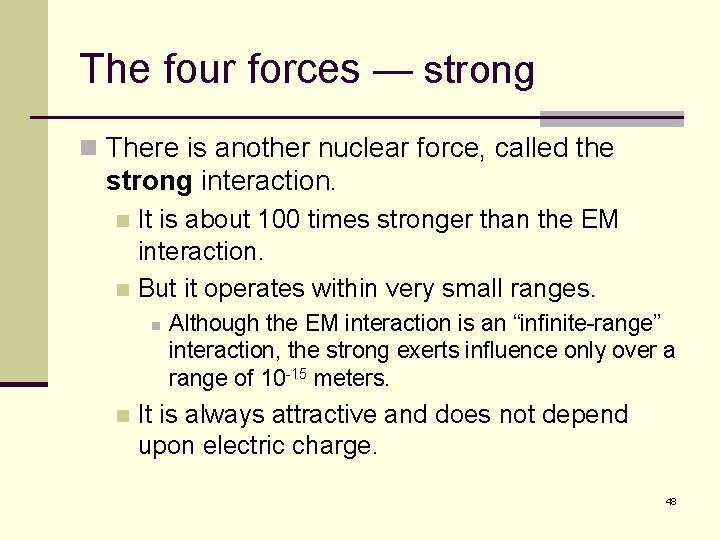
The four forces — strong n There is another nuclear force, called the strong interaction. It is about 100 times stronger than the EM interaction. n But it operates within very small ranges. n n n Although the EM interaction is an “infinite-range” interaction, the strong exerts influence only over a range of 10 -15 meters. It is always attractive and does not depend upon electric charge. 48
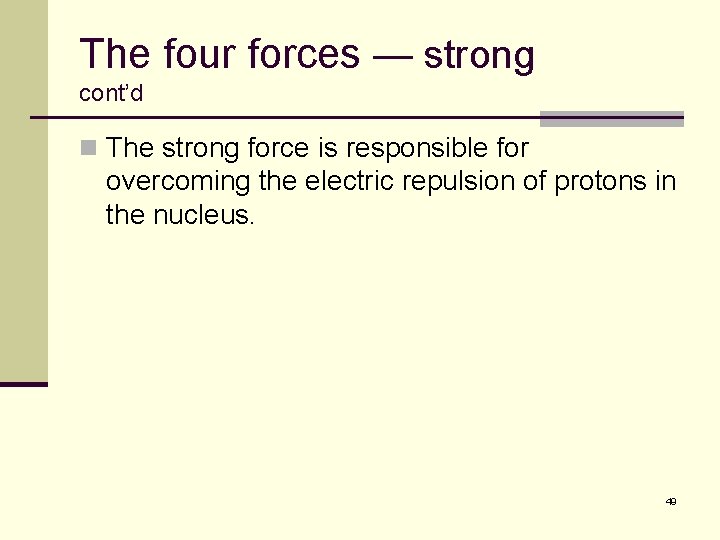
The four forces — strong cont’d n The strong force is responsible for overcoming the electric repulsion of protons in the nucleus. 49
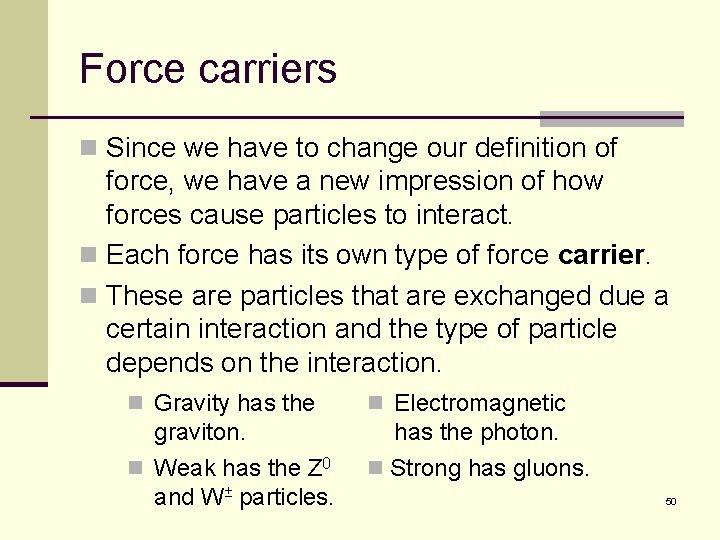
Force carriers n Since we have to change our definition of force, we have a new impression of how forces cause particles to interact. n Each force has its own type of force carrier. n These are particles that are exchanged due a certain interaction and the type of particle depends on the interaction. n Gravity has the graviton. n Weak has the Z 0 and W± particles. n Electromagnetic has the photon. n Strong has gluons. 50
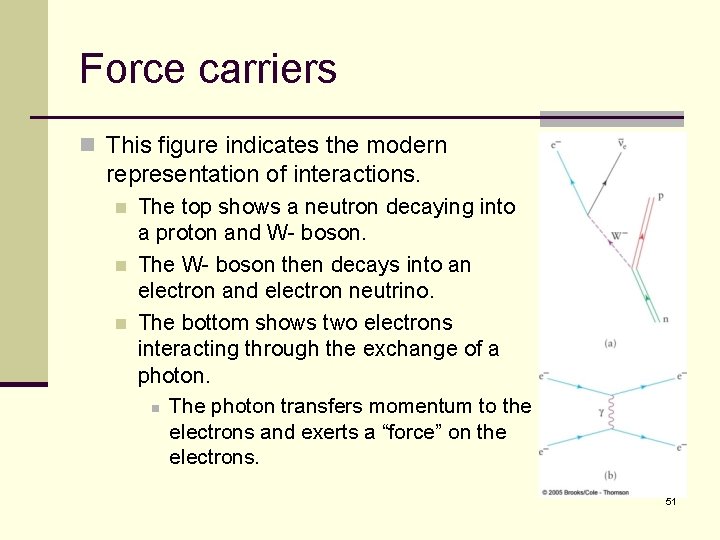
Force carriers n This figure indicates the modern representation of interactions. n n n The top shows a neutron decaying into a proton and W- boson. The W- boson then decays into an electron and electron neutrino. The bottom shows two electrons interacting through the exchange of a photon. n The photon transfers momentum to the electrons and exerts a “force” on the electrons. 51
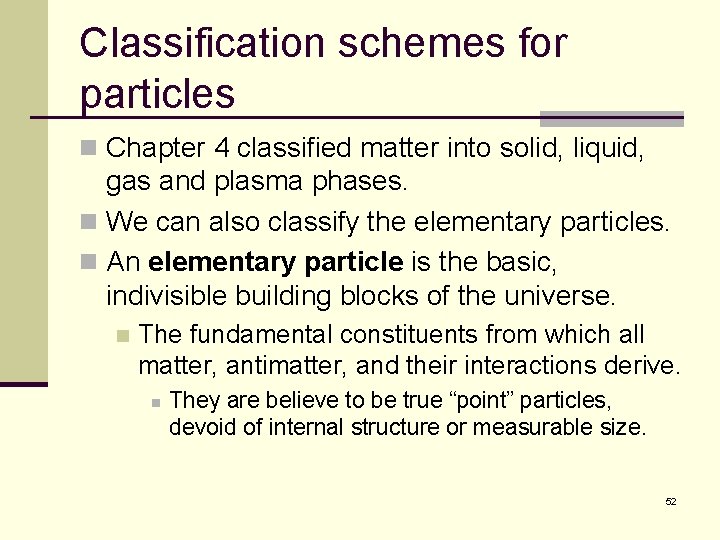
Classification schemes for particles n Chapter 4 classified matter into solid, liquid, gas and plasma phases. n We can also classify the elementary particles. n An elementary particle is the basic, indivisible building blocks of the universe. n The fundamental constituents from which all matter, antimatter, and their interactions derive. n They are believe to be true “point” particles, devoid of internal structure or measurable size. 52
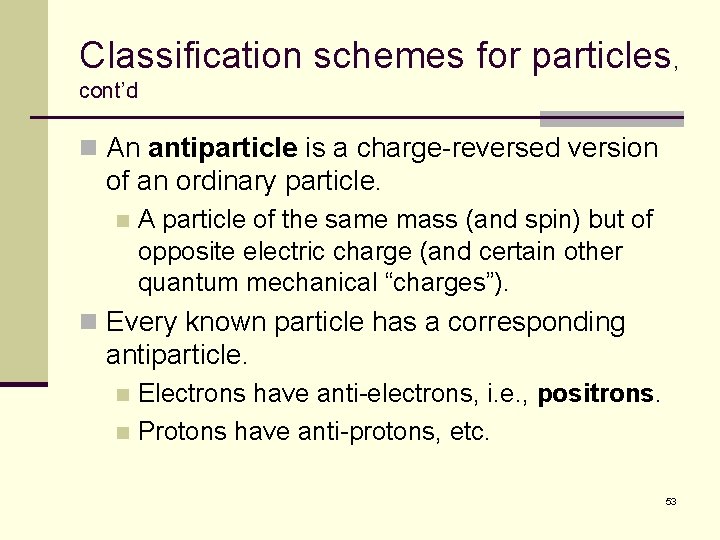
Classification schemes for particles, cont’d n An antiparticle is a charge-reversed version of an ordinary particle. n A particle of the same mass (and spin) but of opposite electric charge (and certain other quantum mechanical “charges”). n Every known particle has a corresponding antiparticle. Electrons have anti-electrons, i. e. , positrons. n Protons have anti-protons, etc. n 53
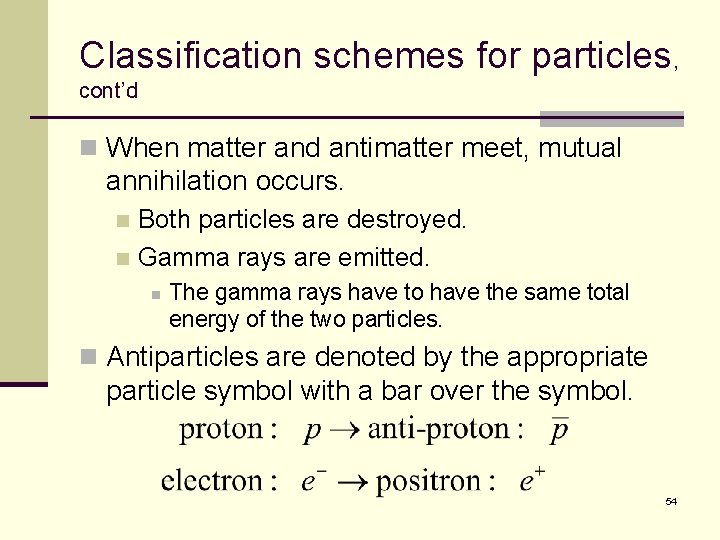
Classification schemes for particles, cont’d n When matter and antimatter meet, mutual annihilation occurs. Both particles are destroyed. n Gamma rays are emitted. n n The gamma rays have to have the same total energy of the two particles. n Antiparticles are denoted by the appropriate particle symbol with a bar over the symbol. 54
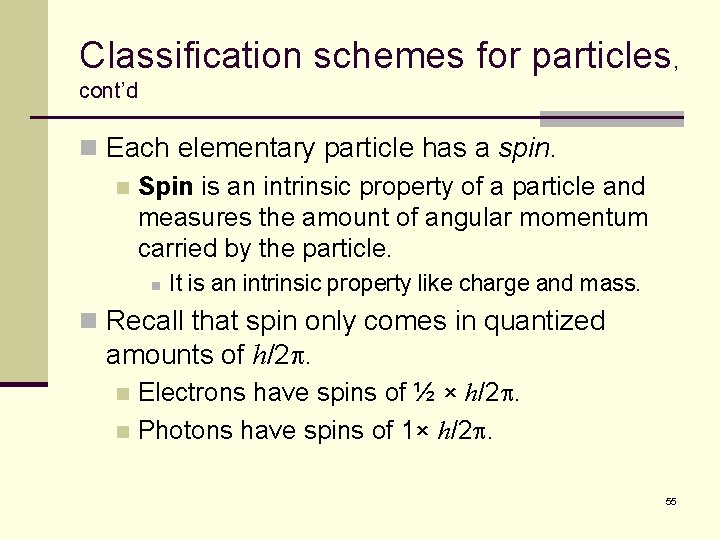
Classification schemes for particles, cont’d n Each elementary particle has a spin. n Spin is an intrinsic property of a particle and measures the amount of angular momentum carried by the particle. n It is an intrinsic property like charge and mass. n Recall that spin only comes in quantized amounts of h/2 p. Electrons have spins of ½ × h/2 p. n Photons have spins of 1× h/2 p. n 55
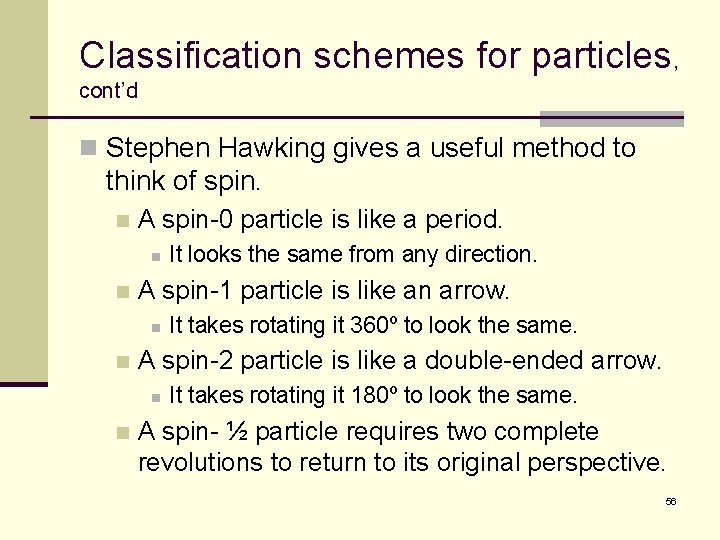
Classification schemes for particles, cont’d n Stephen Hawking gives a useful method to think of spin. n A spin-0 particle is like a period. n n A spin-1 particle is like an arrow. n n It takes rotating it 360º to look the same. A spin-2 particle is like a double-ended arrow. n n It looks the same from any direction. It takes rotating it 180º to look the same. A spin- ½ particle requires two complete revolutions to return to its original perspective. 56
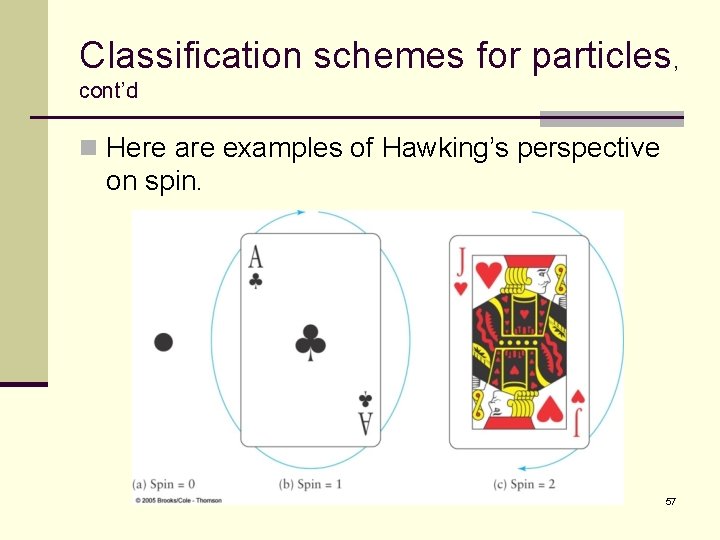
Classification schemes for particles, cont’d n Here are examples of Hawking’s perspective on spin. 57
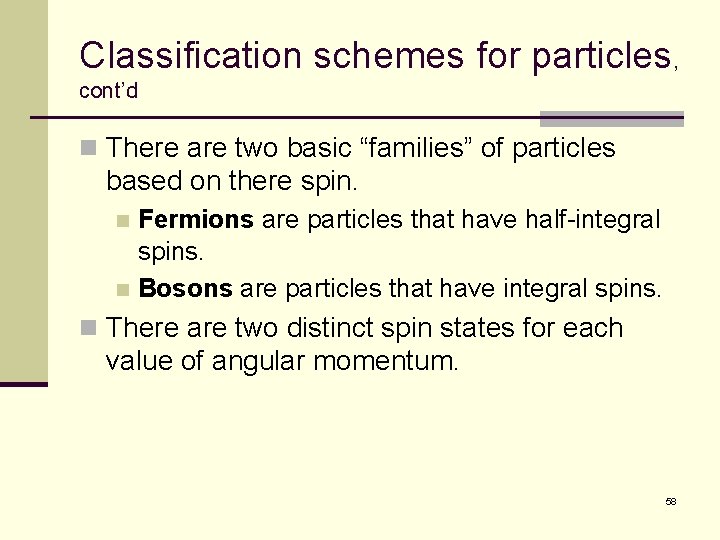
Classification schemes for particles, cont’d n There are two basic “families” of particles based on there spin. Fermions are particles that have half-integral spins. n Bosons are particles that have integral spins. n n There are two distinct spin states for each value of angular momentum. 58
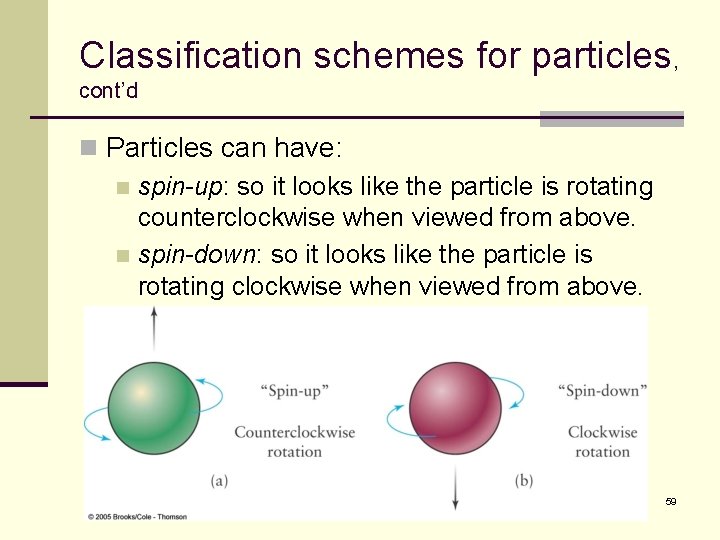
Classification schemes for particles, cont’d n Particles can have: n spin-up: so it looks like the particle is rotating counterclockwise when viewed from above. n spin-down: so it looks like the particle is rotating clockwise when viewed from above. 59
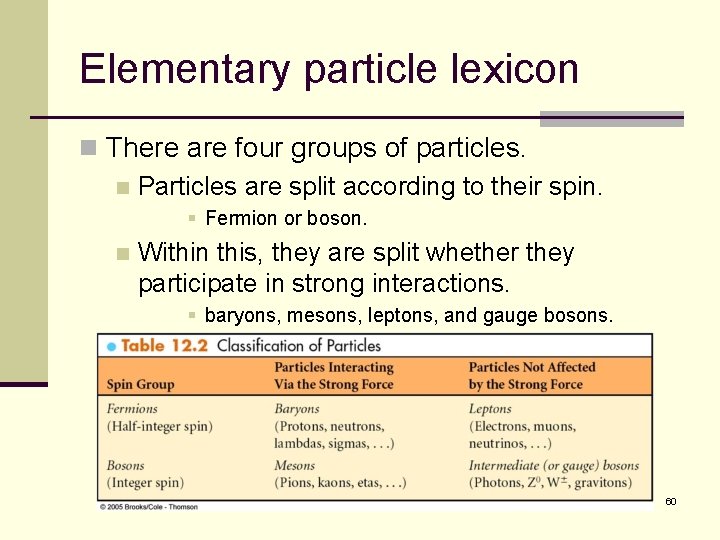
Elementary particle lexicon n There are four groups of particles. n Particles are split according to their spin. § Fermion or boson. n Within this, they are split whether they participate in strong interactions. § baryons, mesons, leptons, and gauge bosons. 60
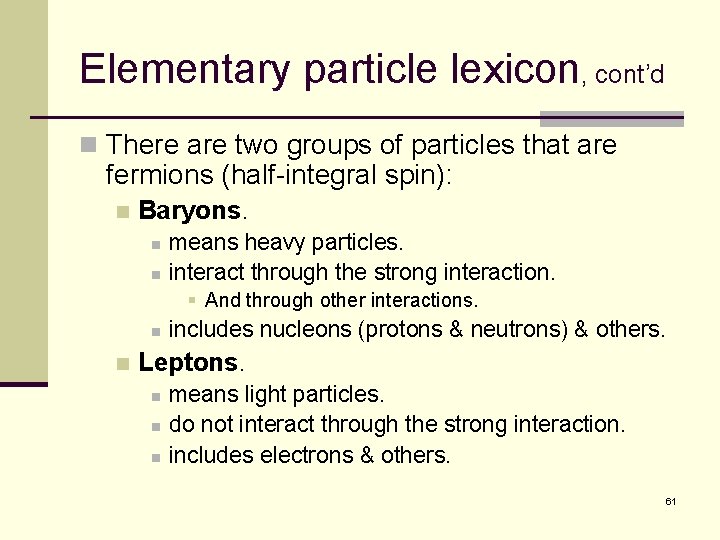
Elementary particle lexicon, cont’d n There are two groups of particles that are fermions (half-integral spin): n Baryons. n n means heavy particles. interact through the strong interaction. § And through other interactions. n n includes nucleons (protons & neutrons) & others. Leptons. n n n means light particles. do not interact through the strong interaction. includes electrons & others. 61
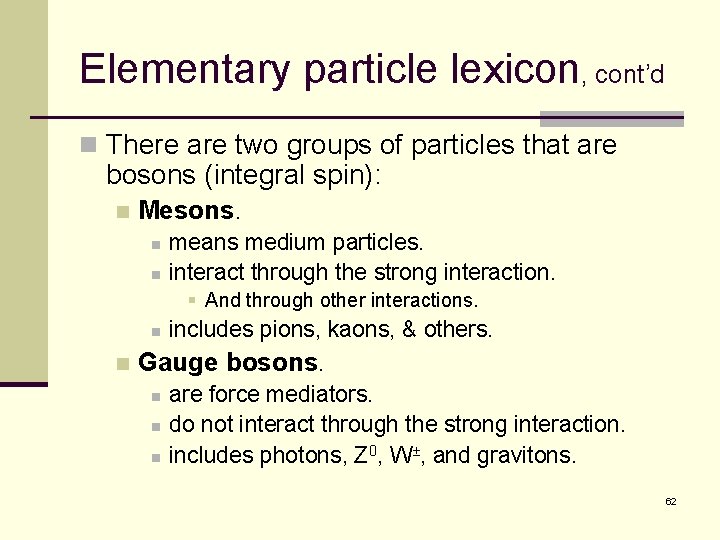
Elementary particle lexicon, cont’d n There are two groups of particles that are bosons (integral spin): n Mesons. n n means medium particles. interact through the strong interaction. § And through other interactions. n n includes pions, kaons, & others. Gauge bosons. n n n are force mediators. do not interact through the strong interaction. includes photons, Z 0, W±, and gravitons. 62
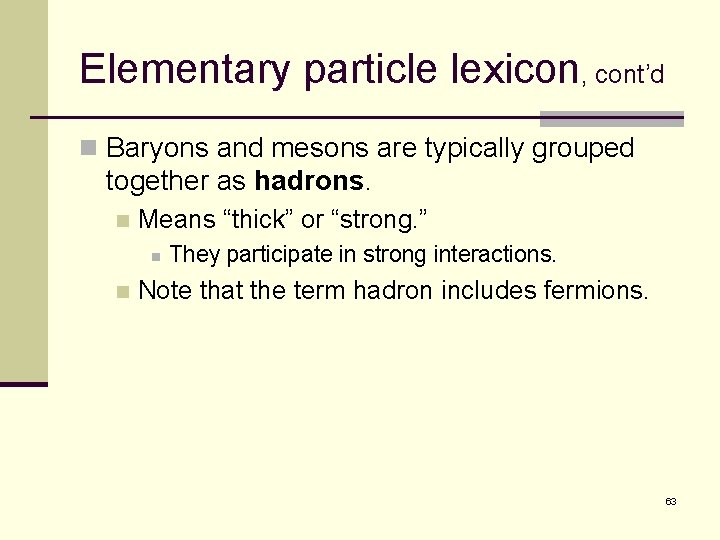
Elementary particle lexicon, cont’d n Baryons and mesons are typically grouped together as hadrons. n Means “thick” or “strong. ” n n They participate in strong interactions. Note that the term hadron includes fermions. 63
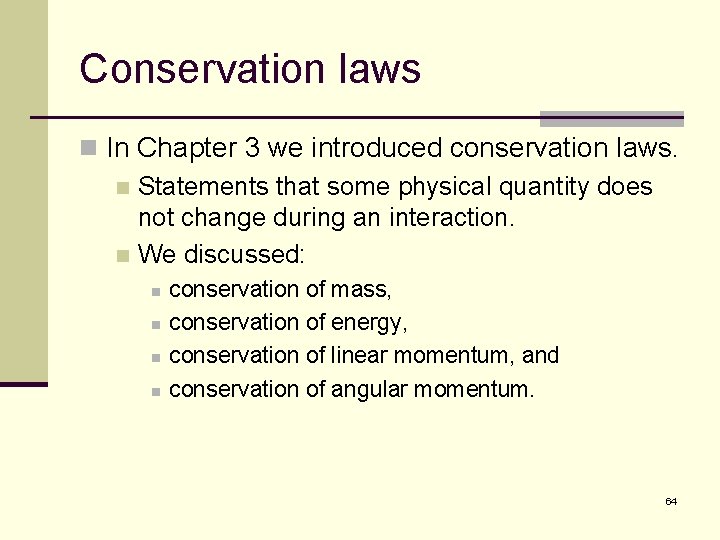
Conservation laws n In Chapter 3 we introduced conservation laws. n Statements that some physical quantity does not change during an interaction. n We discussed: n n conservation of mass, conservation of energy, conservation of linear momentum, and conservation of angular momentum. 64
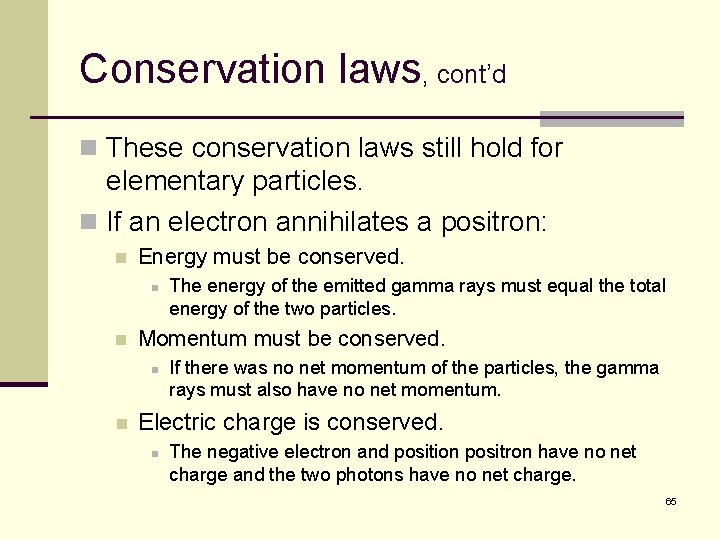
Conservation laws, cont’d n These conservation laws still hold for elementary particles. n If an electron annihilates a positron: n Energy must be conserved. n n Momentum must be conserved. n n The energy of the emitted gamma rays must equal the total energy of the two particles. If there was no net momentum of the particles, the gamma rays must also have no net momentum. Electric charge is conserved. n The negative electron and position positron have no net charge and the two photons have no net charge. 65
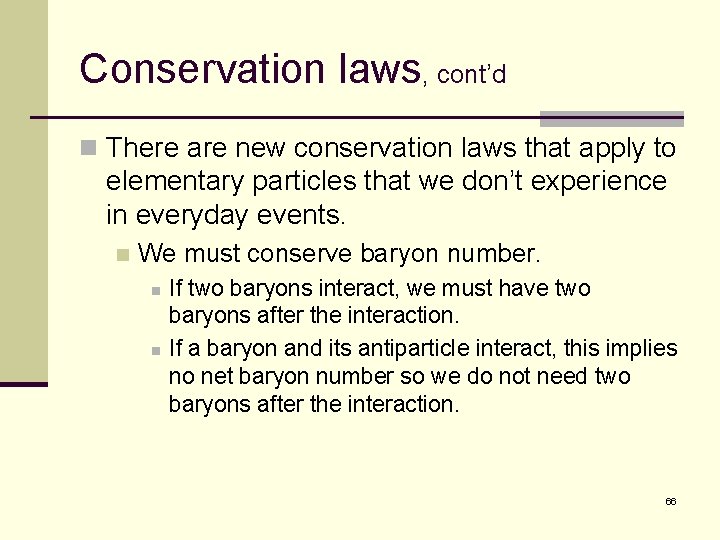
Conservation laws, cont’d n There are new conservation laws that apply to elementary particles that we don’t experience in everyday events. n We must conserve baryon number. n n If two baryons interact, we must have two baryons after the interaction. If a baryon and its antiparticle interact, this implies no net baryon number so we do not need two baryons after the interaction. 66
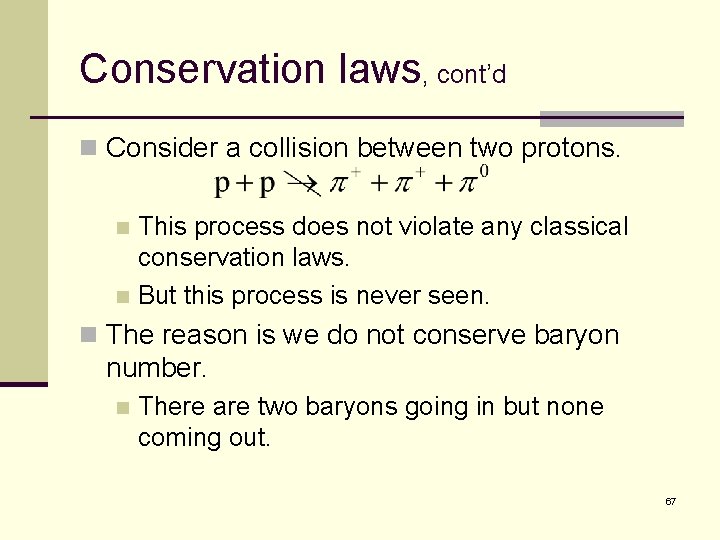
Conservation laws, cont’d n Consider a collision between two protons. This process does not violate any classical conservation laws. n But this process is never seen. n n The reason is we do not conserve baryon number. n There are two baryons going in but none coming out. 67
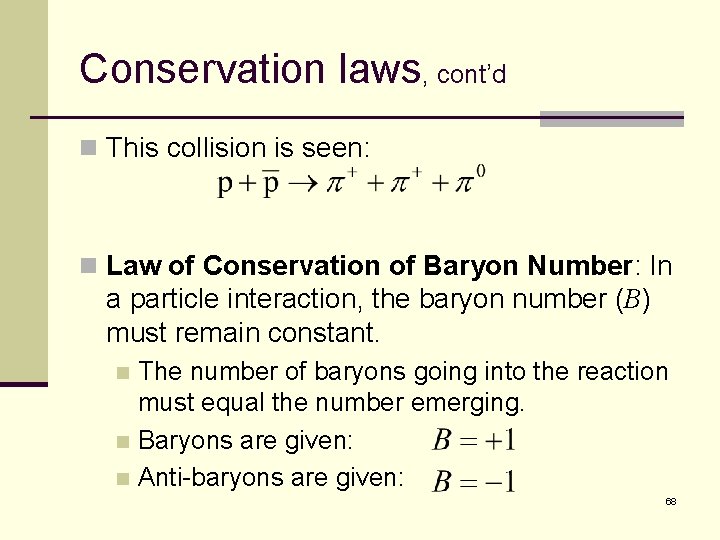
Conservation laws, cont’d n This collision is seen: n Law of Conservation of Baryon Number: In a particle interaction, the baryon number (B) must remain constant. The number of baryons going into the reaction must equal the number emerging. n Baryons are given: n Anti-baryons are given: n 68
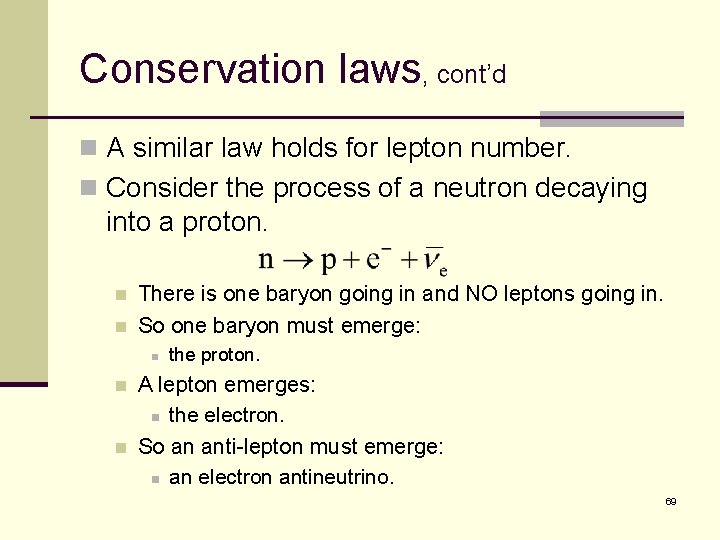
Conservation laws, cont’d n A similar law holds for lepton number. n Consider the process of a neutron decaying into a proton. n n There is one baryon going in and NO leptons going in. So one baryon must emerge: n n n the proton. A lepton emerges: n the electron. So an anti-lepton must emerge: n an electron antineutrino. 69
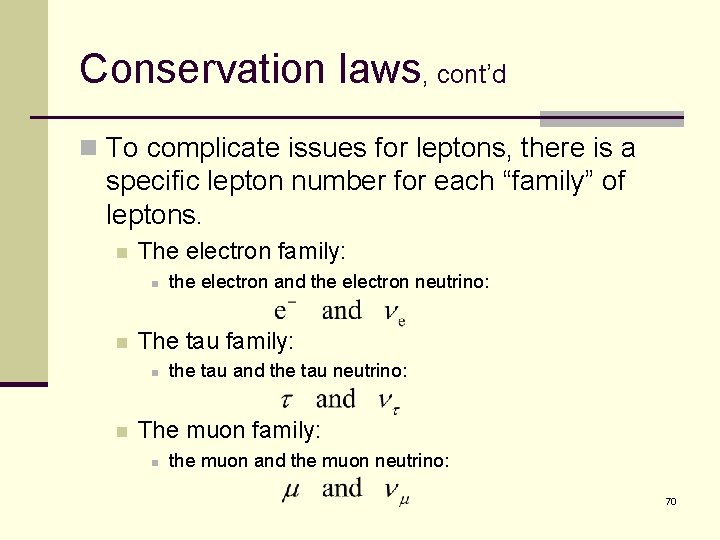
Conservation laws, cont’d n To complicate issues for leptons, there is a specific lepton number for each “family” of leptons. n The electron family: n n The tau family: n n the electron and the electron neutrino: the tau and the tau neutrino: The muon family: n the muon and the muon neutrino: 70
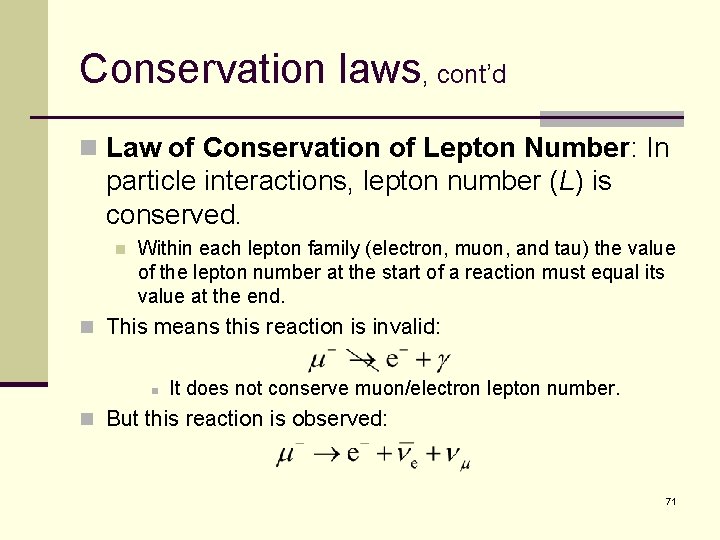
Conservation laws, cont’d n Law of Conservation of Lepton Number: In particle interactions, lepton number (L) is conserved. n Within each lepton family (electron, muon, and tau) the value of the lepton number at the start of a reaction must equal its value at the end. n This means this reaction is invalid: n It does not conserve muon/electron lepton number. n But this reaction is observed: 71
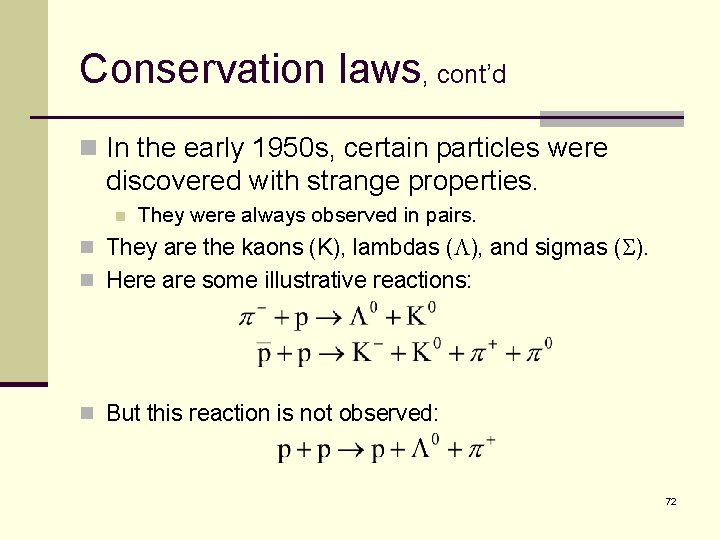
Conservation laws, cont’d n In the early 1950 s, certain particles were discovered with strange properties. n They were always observed in pairs. n They are the kaons (K), lambdas (L), and sigmas (S). n Here are some illustrative reactions: n But this reaction is not observed: 72
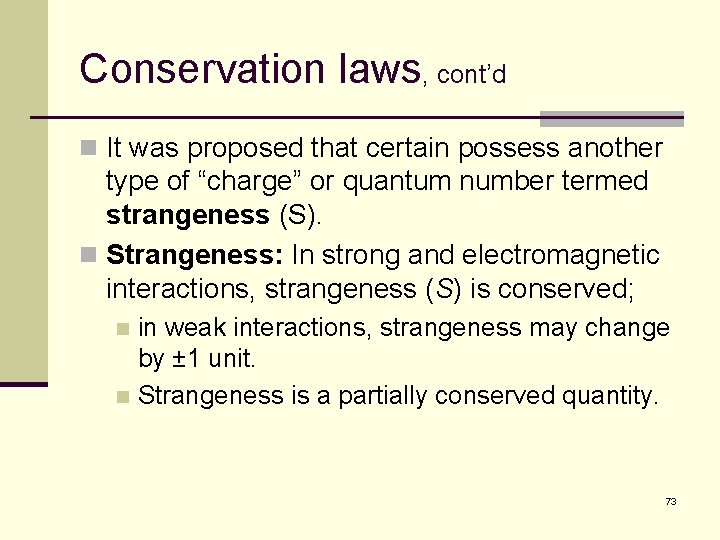
Conservation laws, cont’d n It was proposed that certain possess another type of “charge” or quantum number termed strangeness (S). n Strangeness: In strong and electromagnetic interactions, strangeness (S) is conserved; in weak interactions, strangeness may change by ± 1 unit. n Strangeness is a partially conserved quantity. n 73
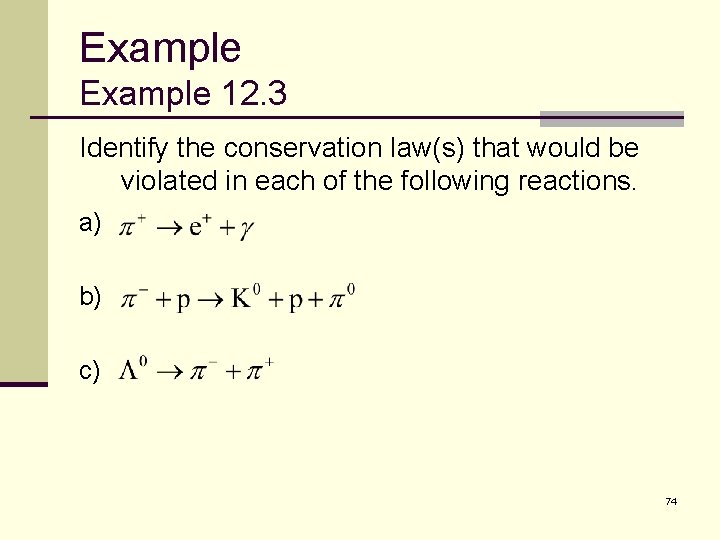
Example 12. 3 Identify the conservation law(s) that would be violated in each of the following reactions. a) b) c) 74
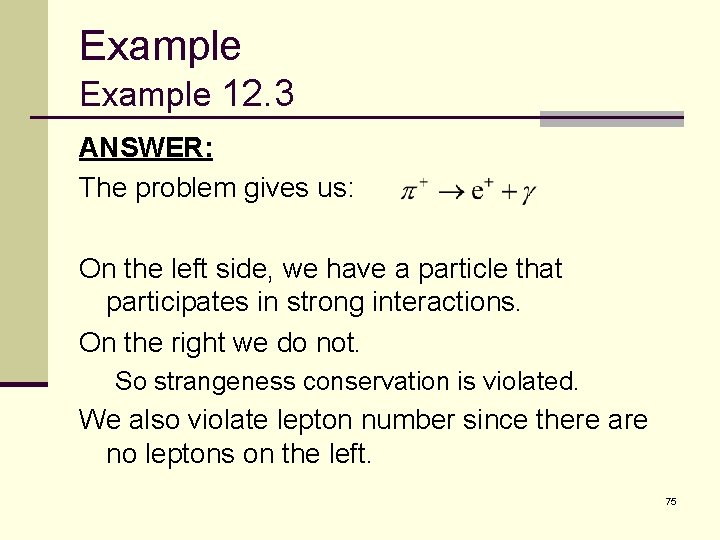
Example 12. 3 ANSWER: The problem gives us: On the left side, we have a particle that participates in strong interactions. On the right we do not. So strangeness conservation is violated. We also violate lepton number since there are no leptons on the left. 75
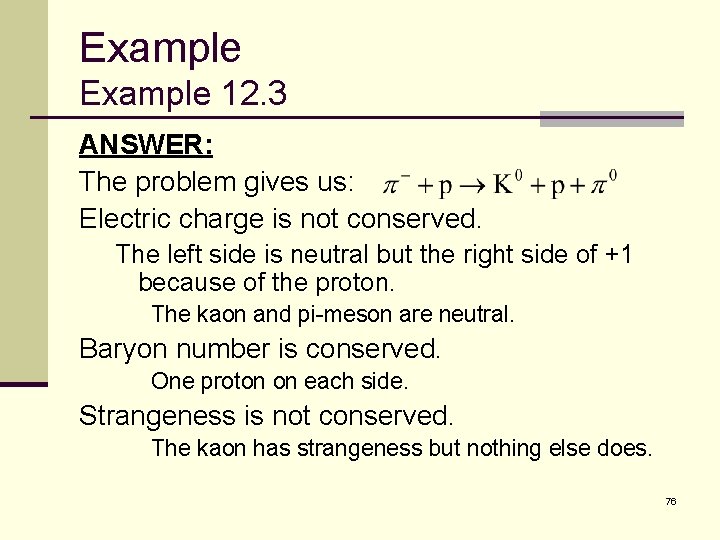
Example 12. 3 ANSWER: The problem gives us: Electric charge is not conserved. The left side is neutral but the right side of +1 because of the proton. The kaon and pi-meson are neutral. Baryon number is conserved. One proton on each side. Strangeness is not conserved. The kaon has strangeness but nothing else does. 76
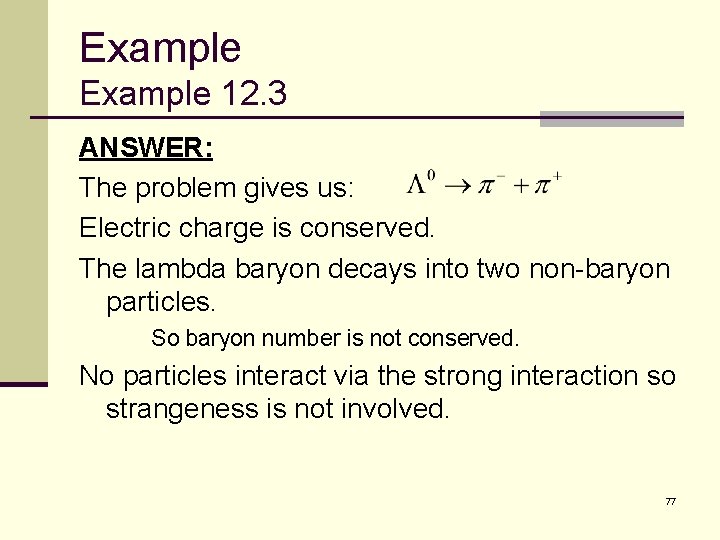
Example 12. 3 ANSWER: The problem gives us: Electric charge is conserved. The lambda baryon decays into two non-baryon particles. So baryon number is not conserved. No particles interact via the strong interaction so strangeness is not involved. 77
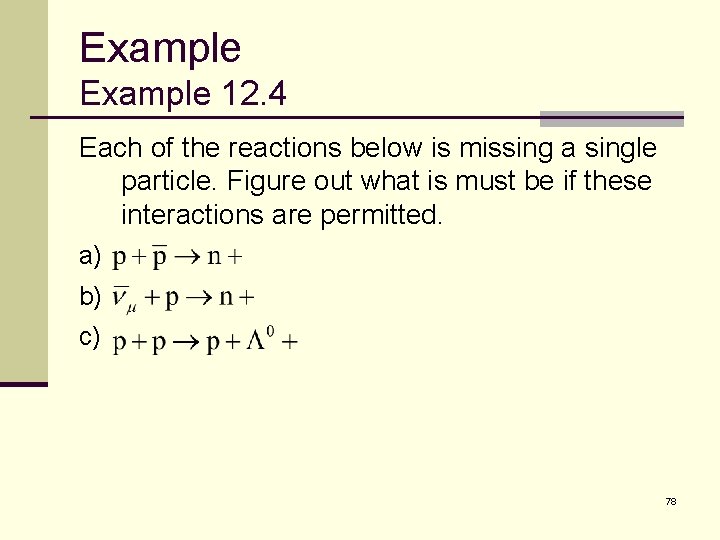
Example 12. 4 Each of the reactions below is missing a single particle. Figure out what is must be if these interactions are permitted. a) b) c) 78
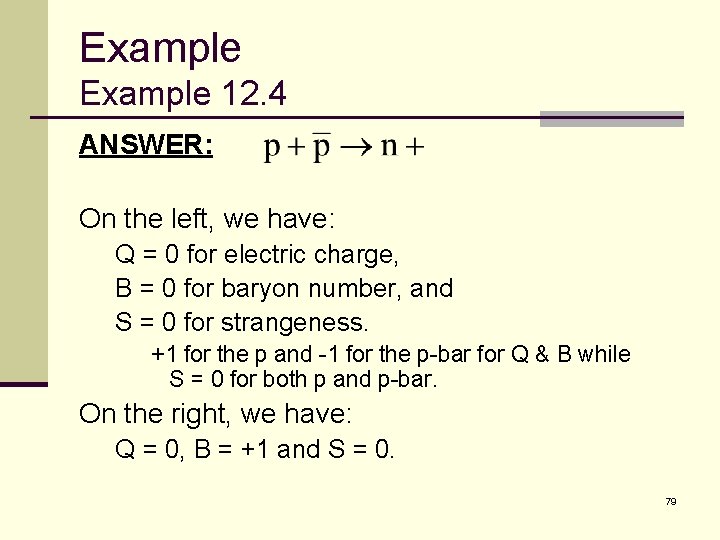
Example 12. 4 ANSWER: On the left, we have: Q = 0 for electric charge, B = 0 for baryon number, and S = 0 for strangeness. +1 for the p and -1 for the p-bar for Q & B while S = 0 for both p and p-bar. On the right, we have: Q = 0, B = +1 and S = 0. 79
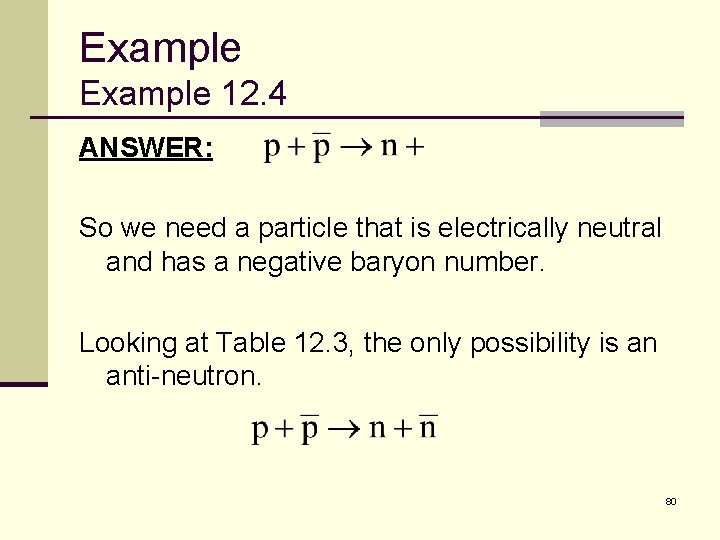
Example 12. 4 ANSWER: So we need a particle that is electrically neutral and has a negative baryon number. Looking at Table 12. 3, the only possibility is an anti-neutron. 80
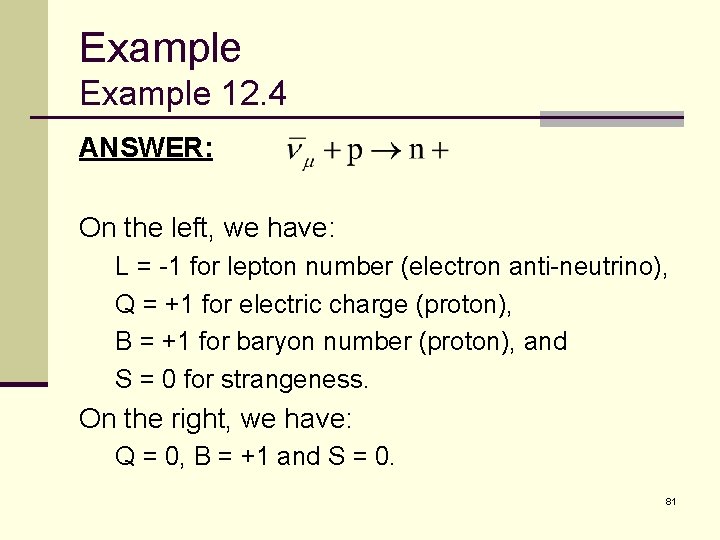
Example 12. 4 ANSWER: On the left, we have: L = -1 for lepton number (electron anti-neutrino), Q = +1 for electric charge (proton), B = +1 for baryon number (proton), and S = 0 for strangeness. On the right, we have: Q = 0, B = +1 and S = 0. 81
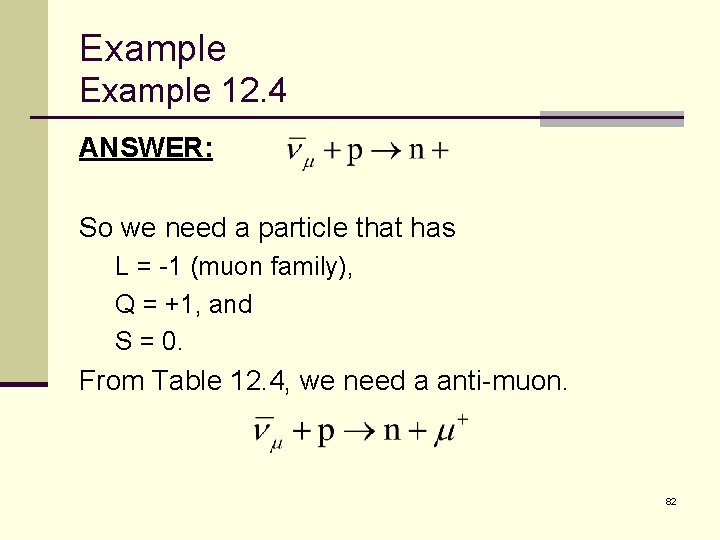
Example 12. 4 ANSWER: So we need a particle that has L = -1 (muon family), Q = +1, and S = 0. From Table 12. 4, we need a anti-muon. 82
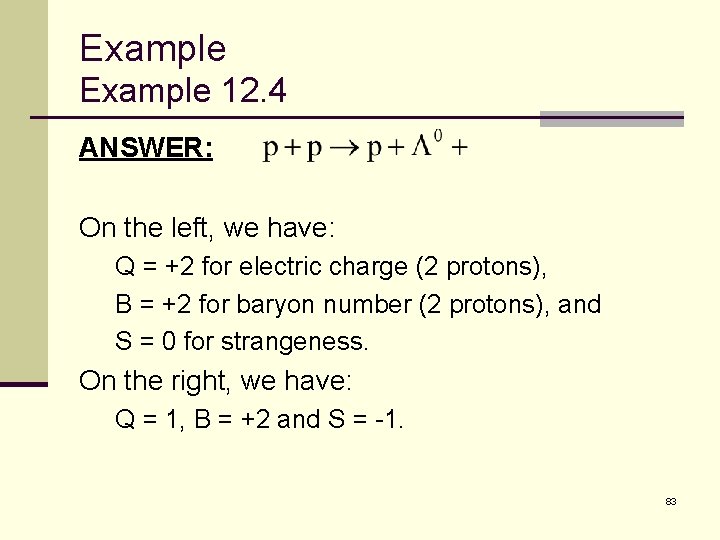
Example 12. 4 ANSWER: On the left, we have: Q = +2 for electric charge (2 protons), B = +2 for baryon number (2 protons), and S = 0 for strangeness. On the right, we have: Q = 1, B = +2 and S = -1. 83
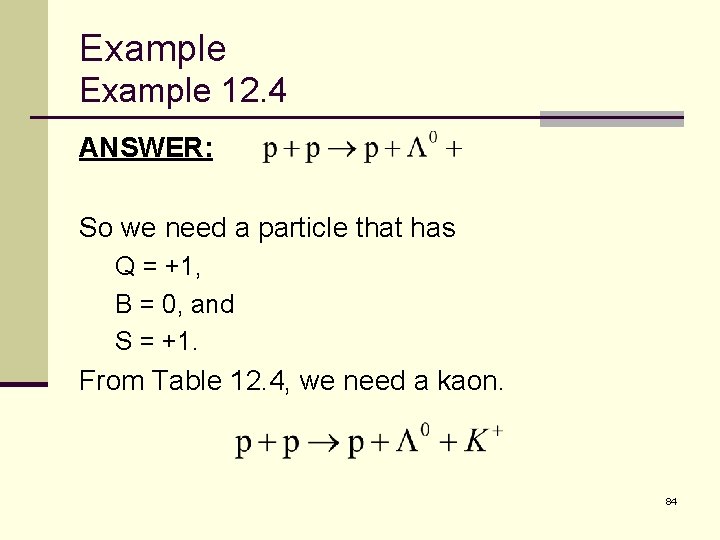
Example 12. 4 ANSWER: So we need a particle that has Q = +1, B = 0, and S = +1. From Table 12. 4, we need a kaon. 84
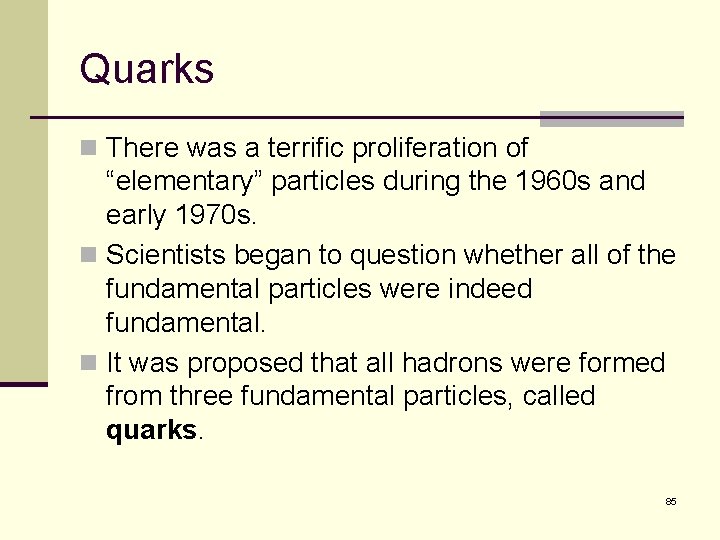
Quarks n There was a terrific proliferation of “elementary” particles during the 1960 s and early 1970 s. n Scientists began to question whether all of the fundamental particles were indeed fundamental. n It was proposed that all hadrons were formed from three fundamental particles, called quarks. 85
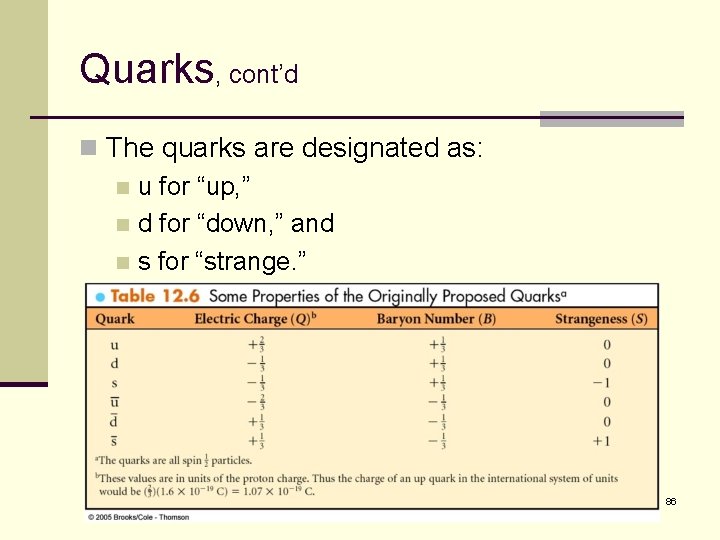
Quarks, cont’d n The quarks are designated as: n u for “up, ” n d for “down, ” and n s for “strange. ” 86
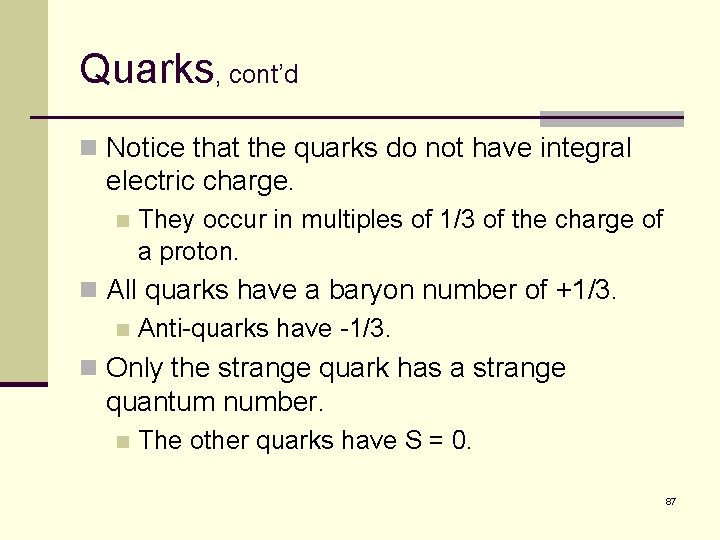
Quarks, cont’d n Notice that the quarks do not have integral electric charge. n They occur in multiples of 1/3 of the charge of a proton. n All quarks have a baryon number of +1/3. n Anti-quarks have -1/3. n Only the strange quark has a strange quantum number. n The other quarks have S = 0. 87
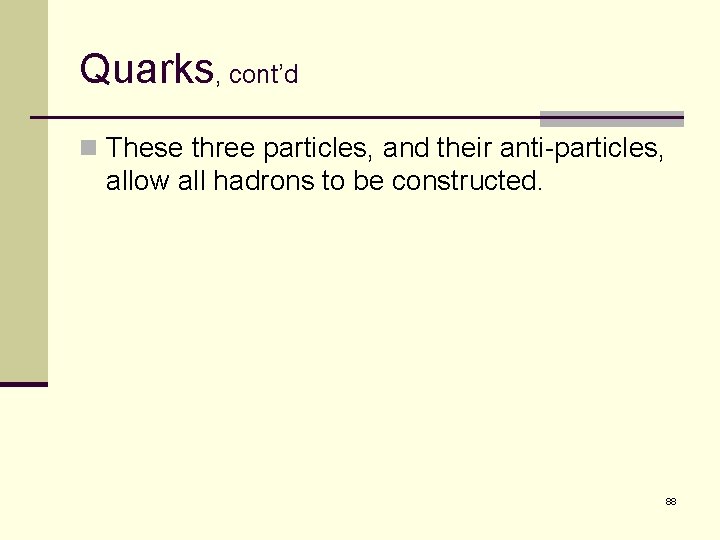
Quarks, cont’d n These three particles, and their anti-particles, allow all hadrons to be constructed. 88
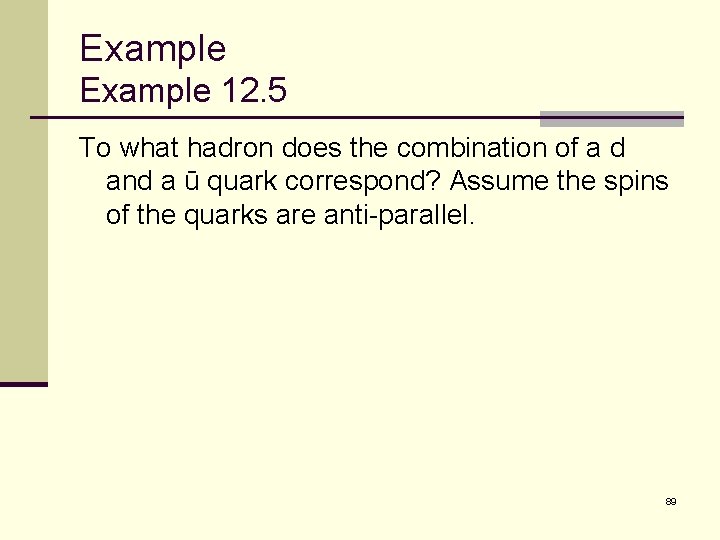
Example 12. 5 To what hadron does the combination of a d and a ū quark correspond? Assume the spins of the quarks are anti-parallel. 89
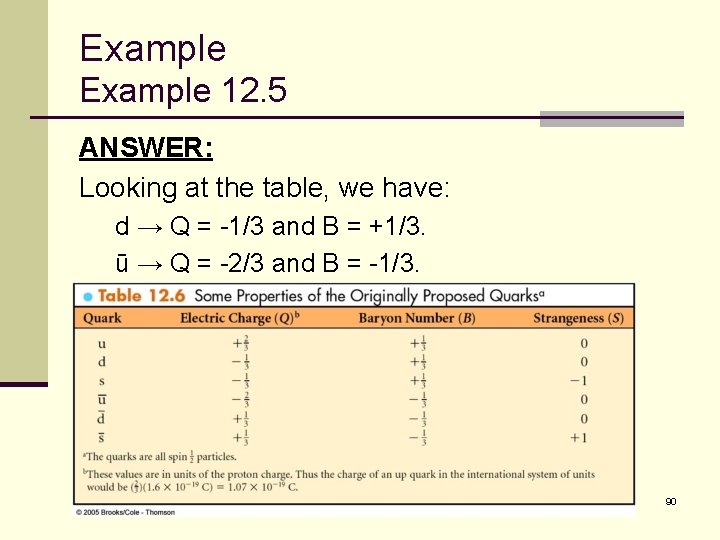
Example 12. 5 ANSWER: Looking at the table, we have: d → Q = -1/3 and B = +1/3. ū → Q = -2/3 and B = -1/3. 90
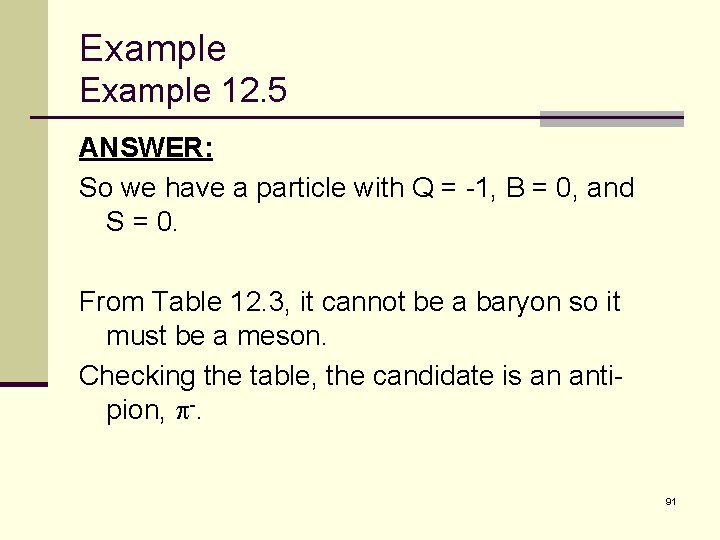
Example 12. 5 ANSWER: So we have a particle with Q = -1, B = 0, and S = 0. From Table 12. 3, it cannot be a baryon so it must be a meson. Checking the table, the candidate is an antipion, p-. 91
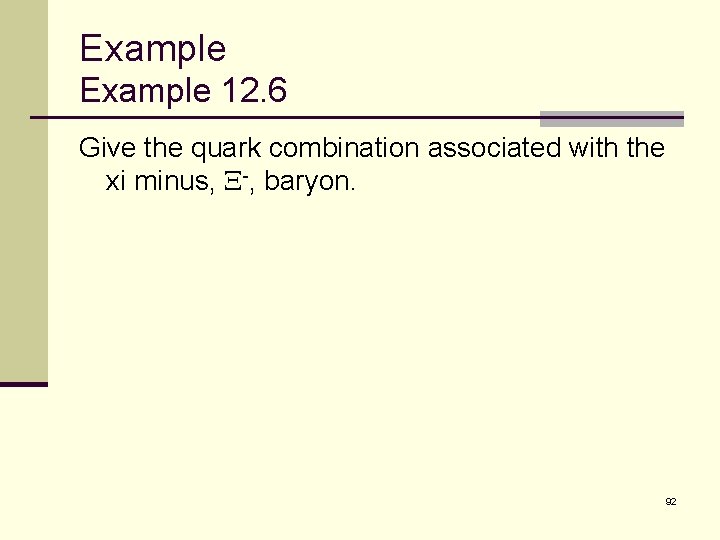
Example 12. 6 Give the quark combination associated with the xi minus, X-, baryon. 92
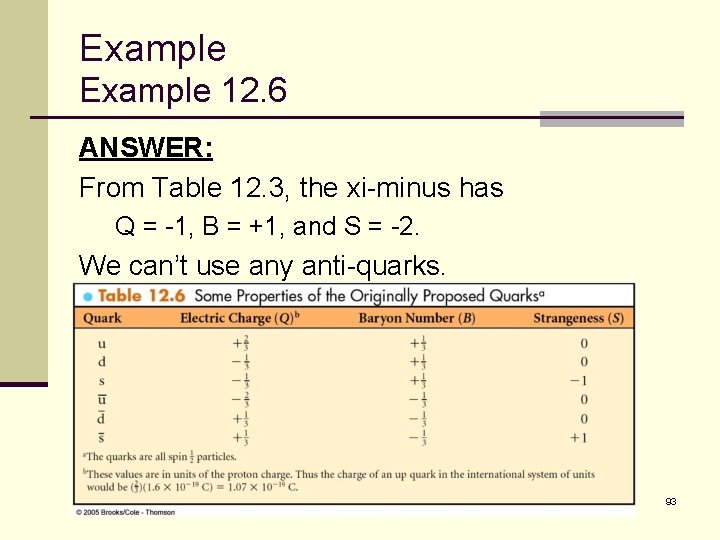
Example 12. 6 ANSWER: From Table 12. 3, the xi-minus has Q = -1, B = +1, and S = -2. We can’t use any anti-quarks. 93
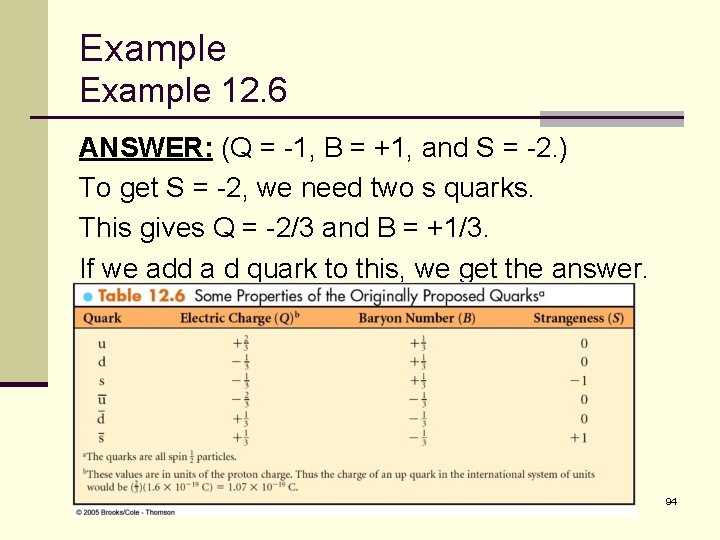
Example 12. 6 ANSWER: (Q = -1, B = +1, and S = -2. ) To get S = -2, we need two s quarks. This gives Q = -2/3 and B = +1/3. If we add a d quark to this, we get the answer. 94
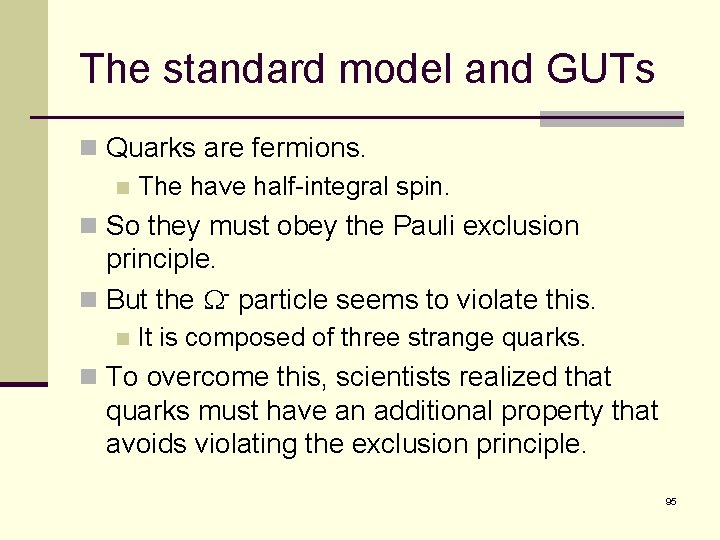
The standard model and GUTs n Quarks are fermions. n The have half-integral spin. n So they must obey the Pauli exclusion principle. n But the W- particle seems to violate this. n It is composed of three strange quarks. n To overcome this, scientists realized that quarks must have an additional property that avoids violating the exclusion principle. 95
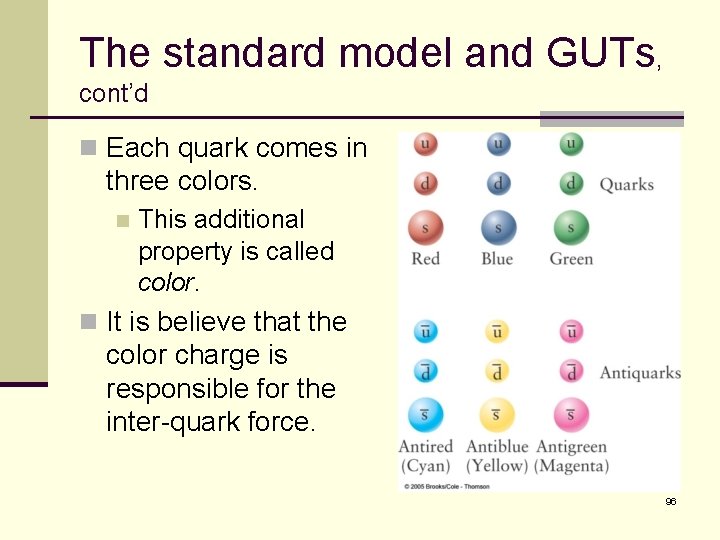
The standard model and GUTs, cont’d n Each quark comes in three colors. n This additional property is called color. n It is believe that the color charge is responsible for the inter-quark force. 96
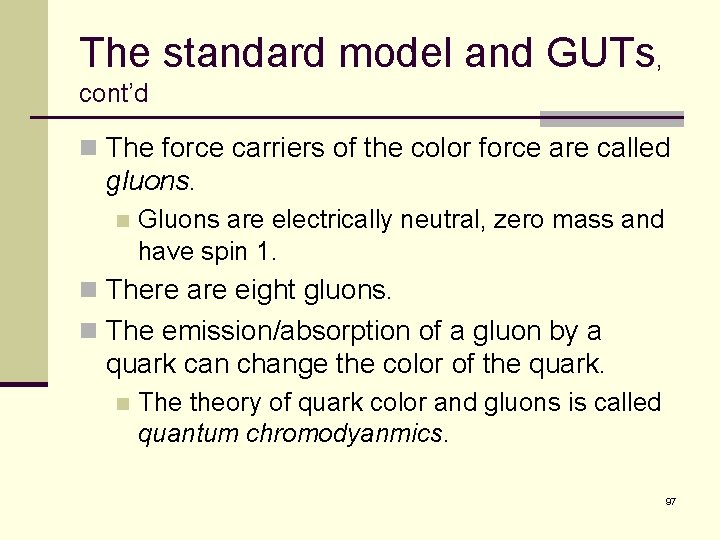
The standard model and GUTs, cont’d n The force carriers of the color force are called gluons. n Gluons are electrically neutral, zero mass and have spin 1. n There are eight gluons. n The emission/absorption of a gluon by a quark can change the color of the quark. n The theory of quark color and gluons is called quantum chromodyanmics. 97
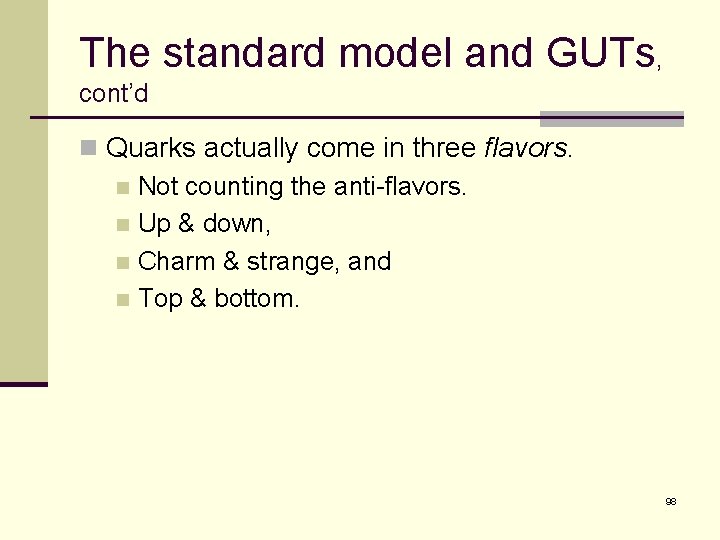
The standard model and GUTs, cont’d n Quarks actually come in three flavors. n Not counting the anti-flavors. n Up & down, n Charm & strange, and n Top & bottom. 98
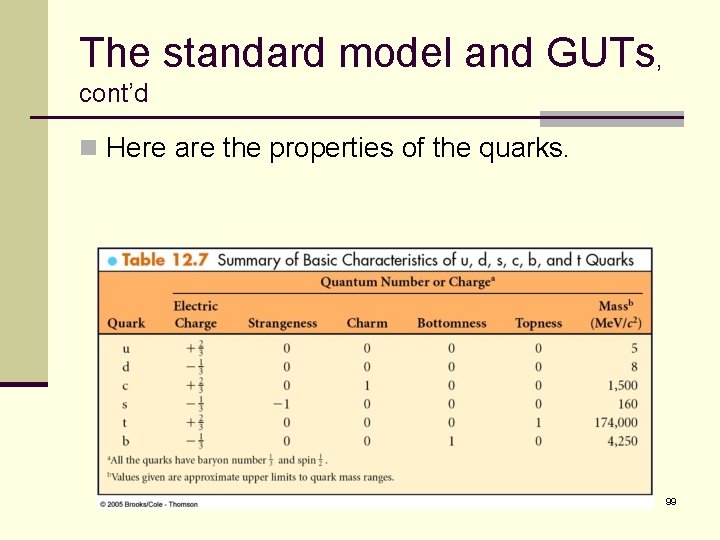
The standard model and GUTs, cont’d n Here are the properties of the quarks. 99
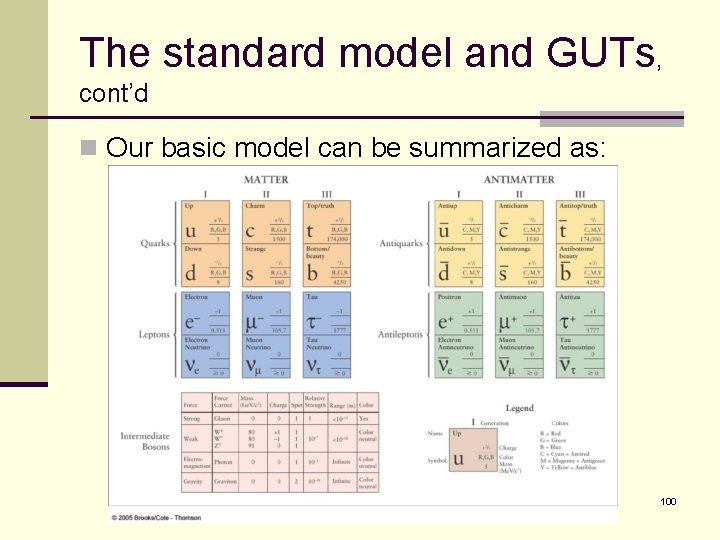
The standard model and GUTs, cont’d n Our basic model can be summarized as: 100
Boost interprocess
General relativity vs special relativity
General relativity vs special relativity
Special relativity
What is special relativity
Postulate
Modern physics means
Momentum special relativity
Special relativity higher physics
Special relativity summary
Albert einstein theory of special relativity
Postulates of special theory of relativity
Postulates of special theory of relativity
Special relativity
Special relativity
Eddington
Special relativity for dummies
Classification of elementary particle
Classification of elementary particles
Ions charged particles in solution
Theory of relativity
Linguistic relativity definition
Relativity for dummies
Relativity definition
General relativity equation
Sapir whorf hopi
Newtonian relativity
Time dilation presentation
Hridis pal
Relativity
Galilean relativity
Time relativity interstellar
Principle of linguistic relativity
Lorentz transformation equation
Relativity
Definition of linguistic determinism
Careershodh
Theory of relativity cartoon
Galileo's principle of relativity
Definition of linguistic determinism
General theory of relativity
The linguistic relativity hypothesis
Law of relativity
Cultural relativism examples
"relativity"
Art of combining spoken and written words
I language
Relativity str
Theory of general relativity
Gas law relationships
Arrangement particles of liquid
Properties of solids and liquids
35 - 17
Atom and subatomic particles
Fundamental particles and interactions
Motion of particles in solids, liquids and gases
On heating solids
Christian schill
Particles table
Connected particles a level maths
Mass of subatomic particles in amu
Symbol for relative
How do particles move in a liquid
Kinetic theory of solids
Solid
Solid spherical particles of coffee extract from a dryer
The search for fractionally charged particles has–
Solid liquid and gas diagram
Photochromic particles
Charged particles can be accelerated by
Plasma particle arrangement
Buoyancyability
Point like particles
Kmt postulate size
Loose solid particles
Arithmetic mean diameter formula
Is vaporization endo or exothermic
Subatomic particles table
How are particles arranged in an atom
Atoms are small,hard particles
Heterogeneous mixture of intermediate size particles
Spaces between particles of liquid
Subatomic particles symbols
Relative motion of two particles using translating axes
Virtual particles
Subatomic particles description
A cloudy liquid that contains insoluble particles
Subatomic particles description
Particles in motion
Rectilinear motion of particles
Katakana particles
Atoms seldom exist as independent particles
Cloudy mixture with particles that move erratically
How do particles behave
Kinetics of particles newton's second law
Benjamin schumacher
Atoms seldom exist as independent particles
Lewis structure for c2hcl
Subatomic particles table
Subatomic particles can usually pass undeflected
Building block of matter which contains subatomic particles