Chapter 10 Photosynthesis Autotrophs and Heterotrophs Autotrophs are
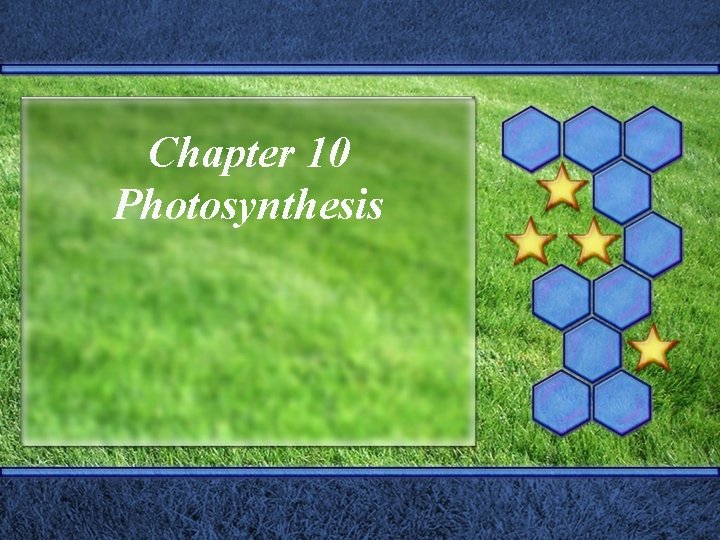
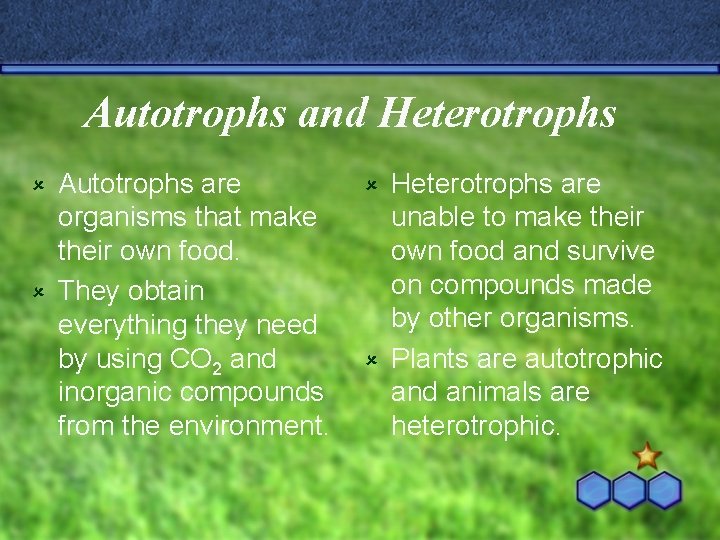
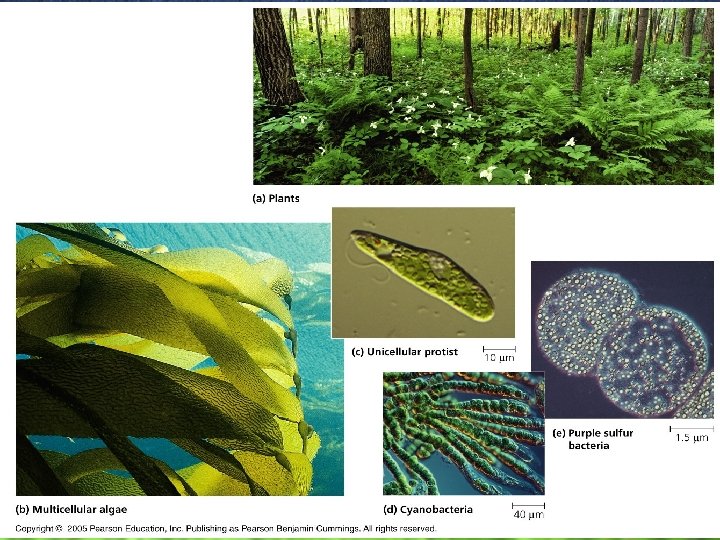
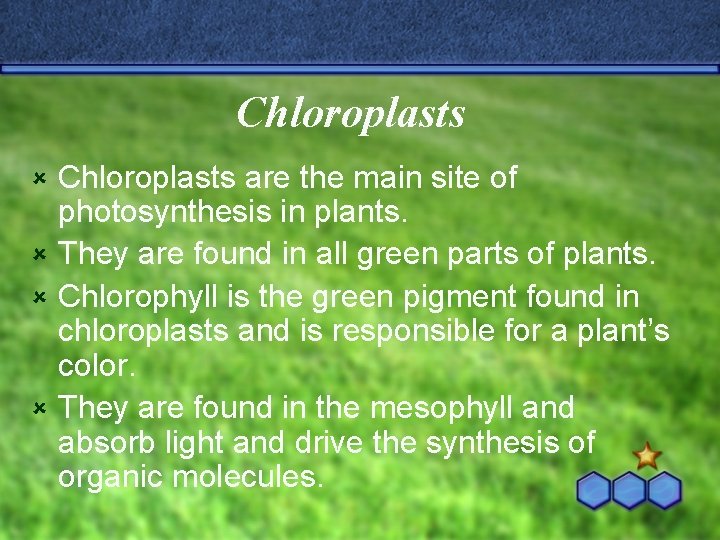
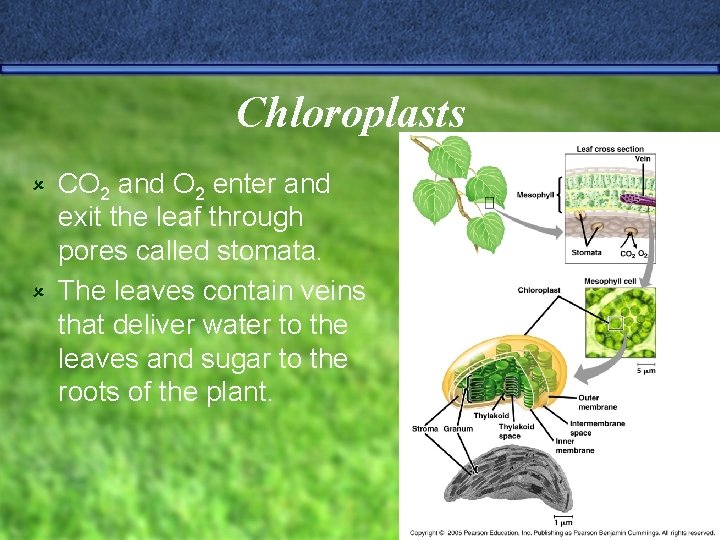
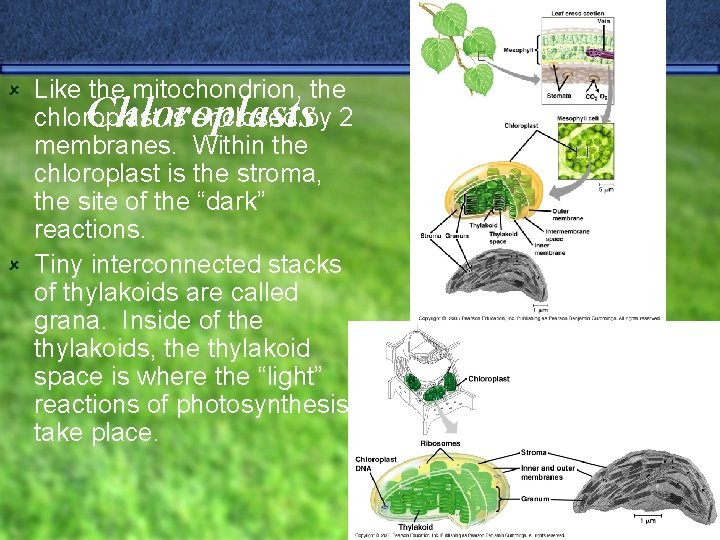
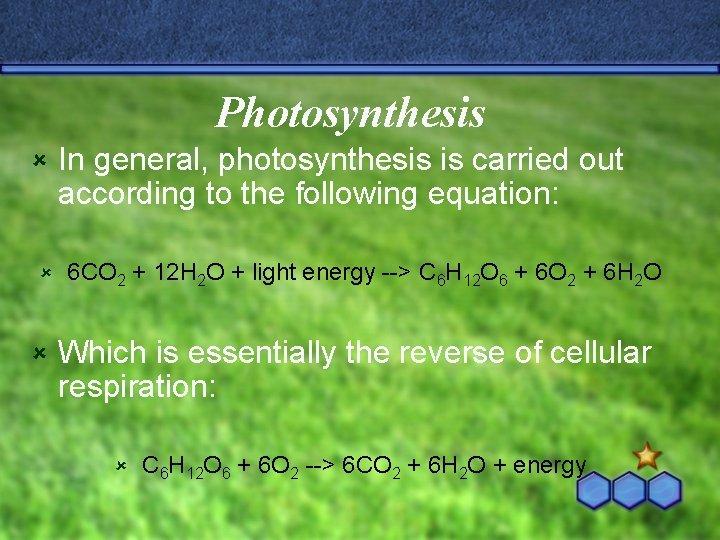
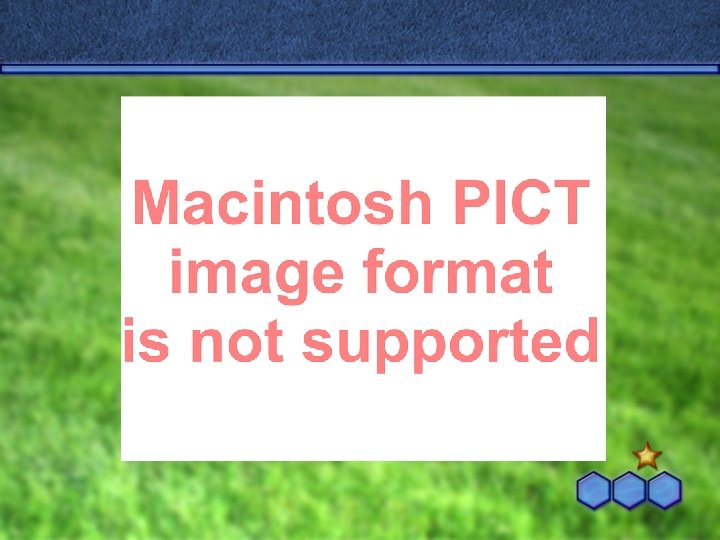
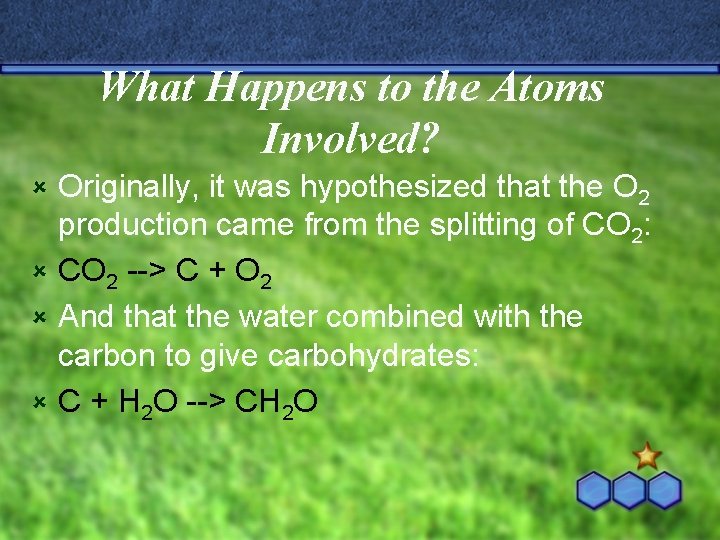
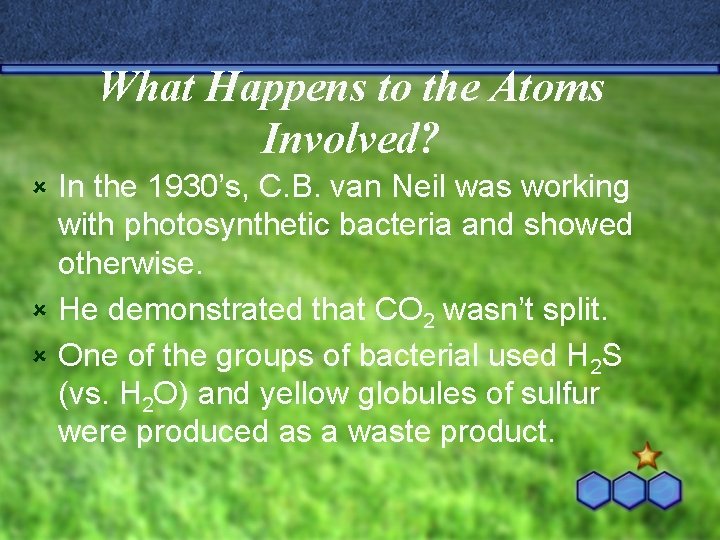
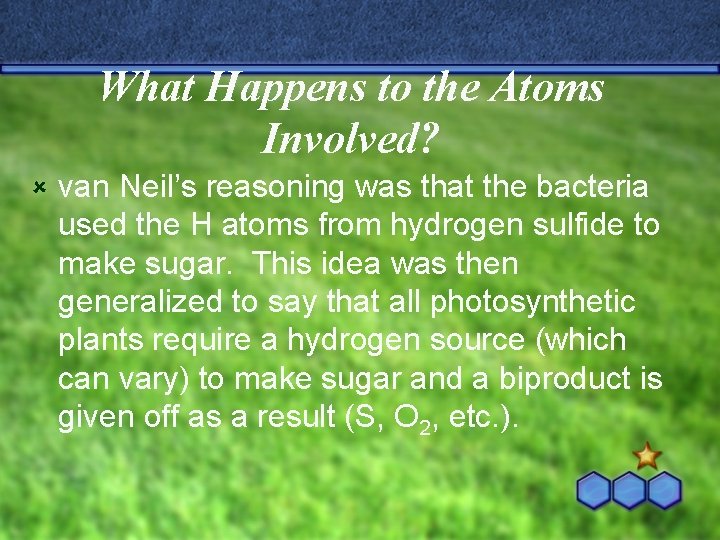
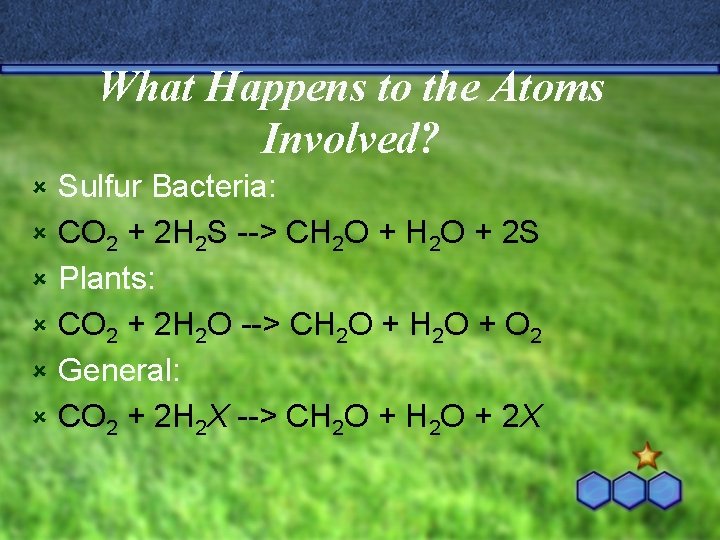
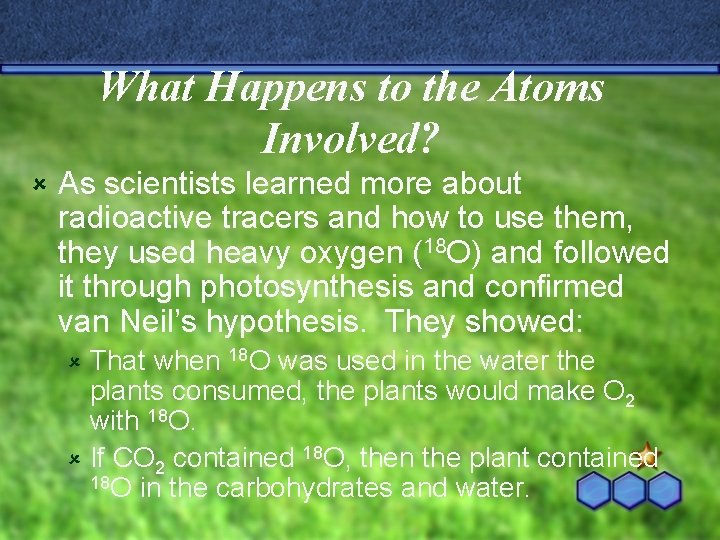
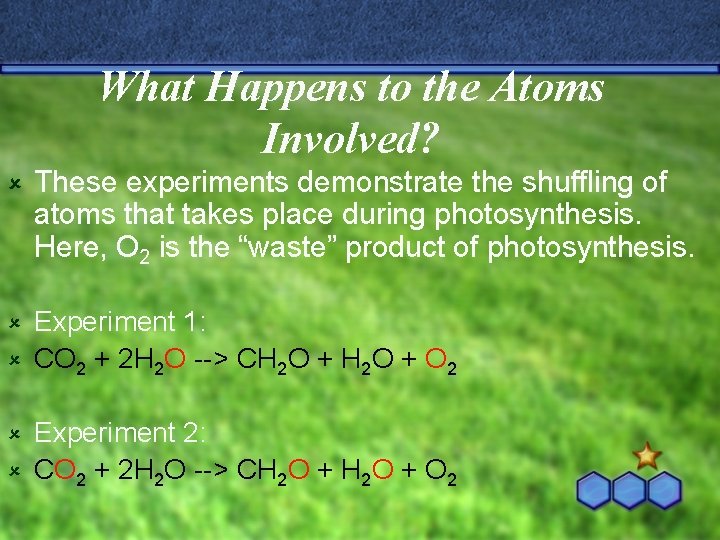
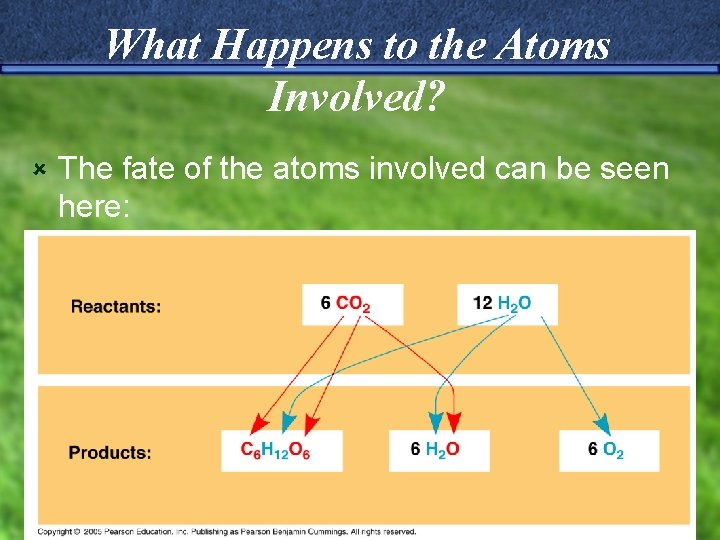
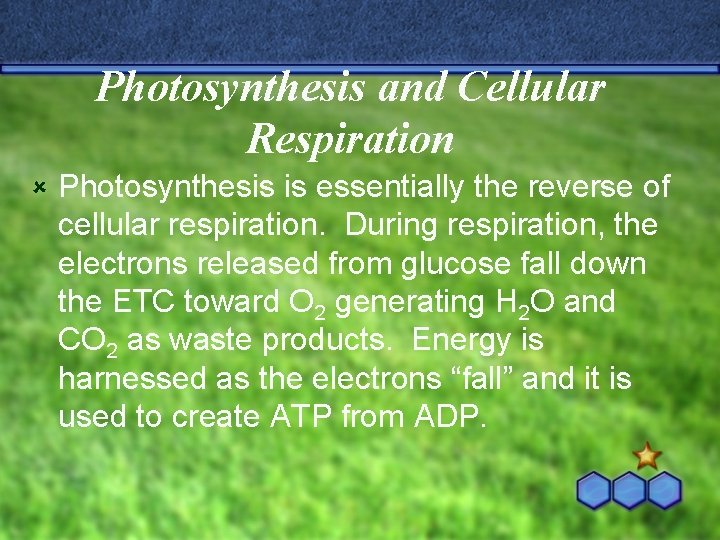
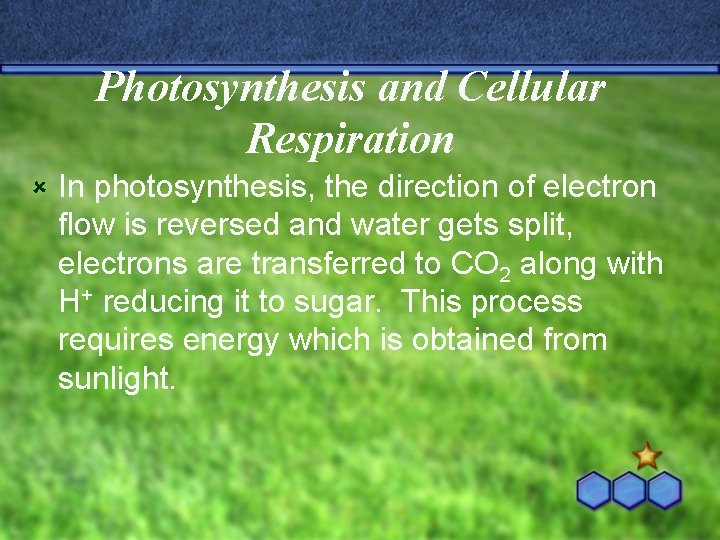
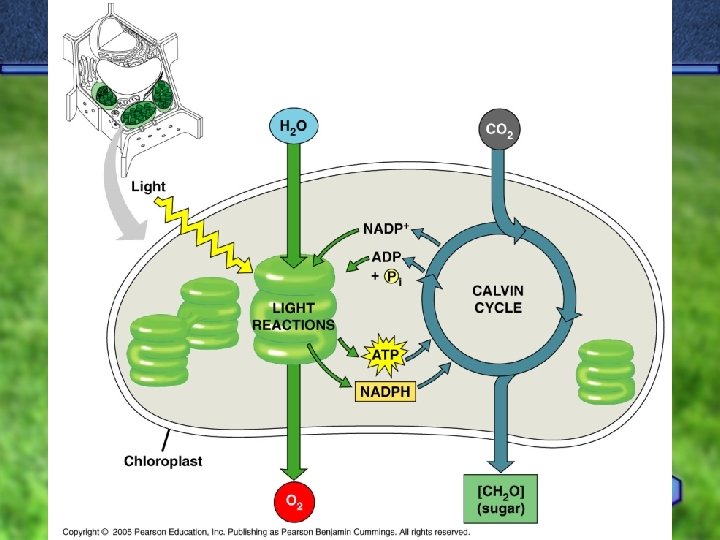
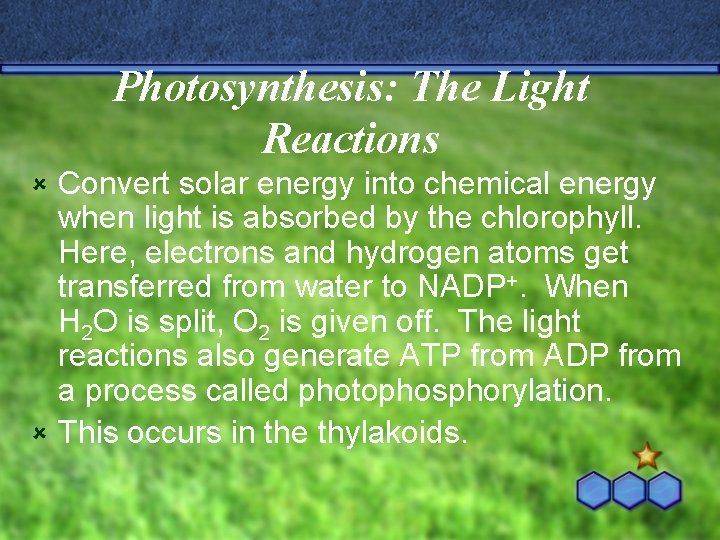
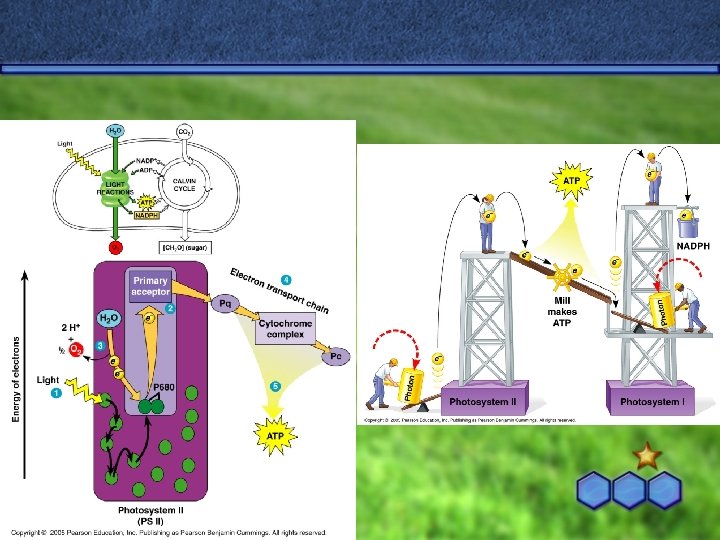
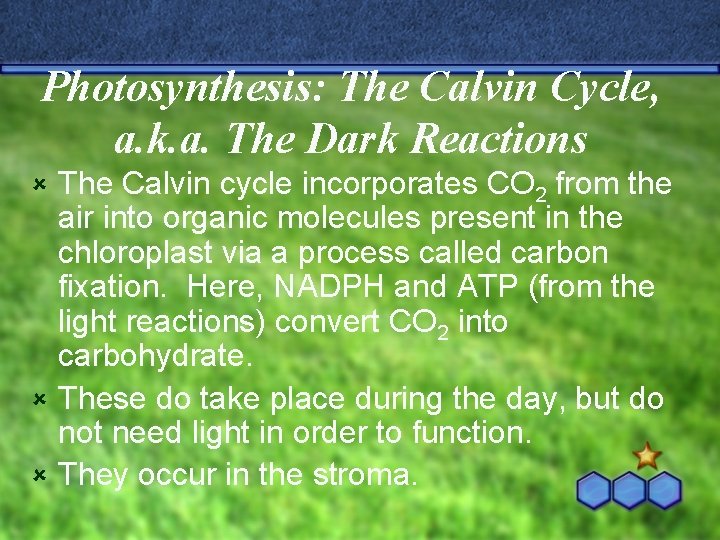
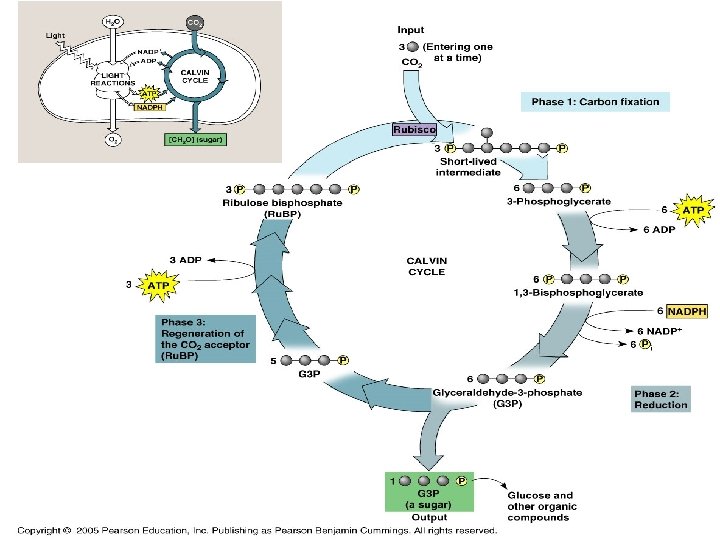
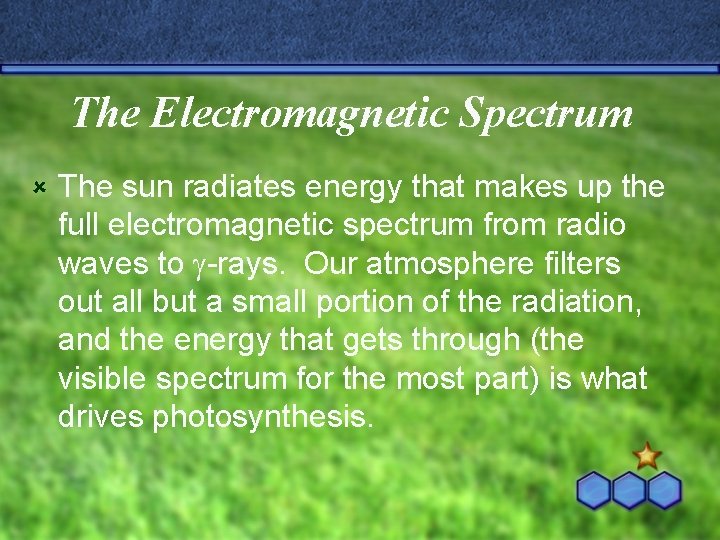
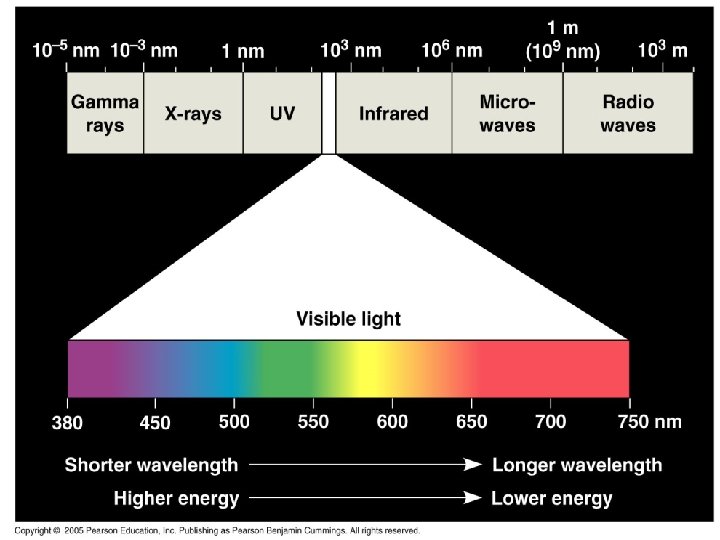
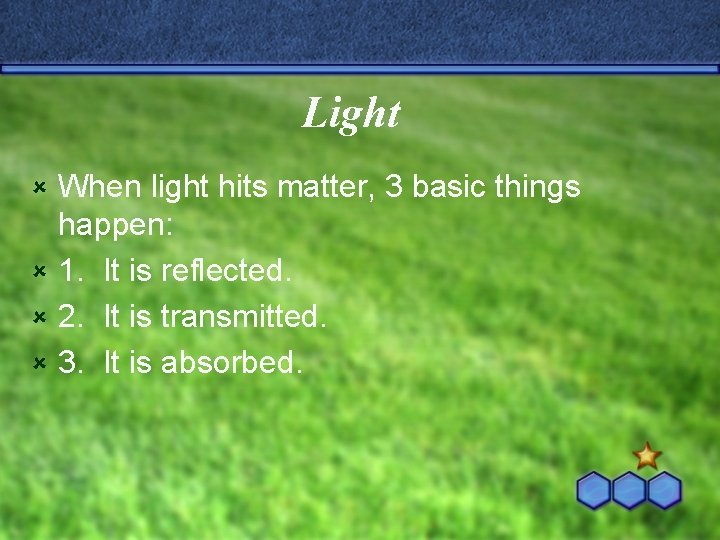
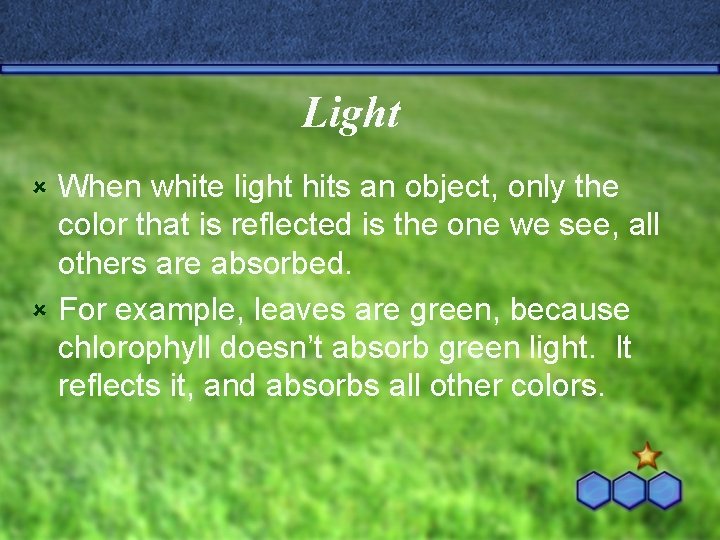
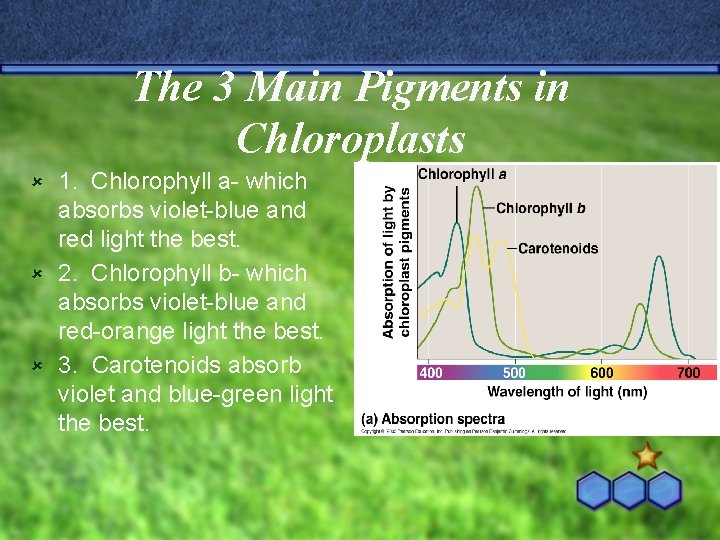
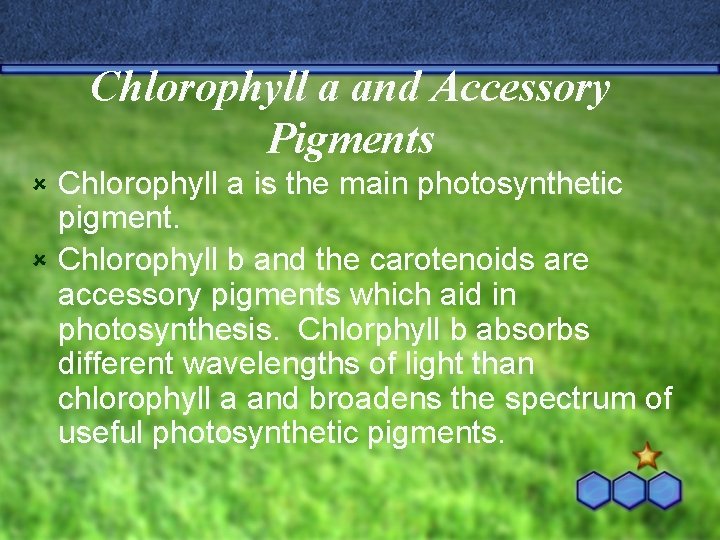
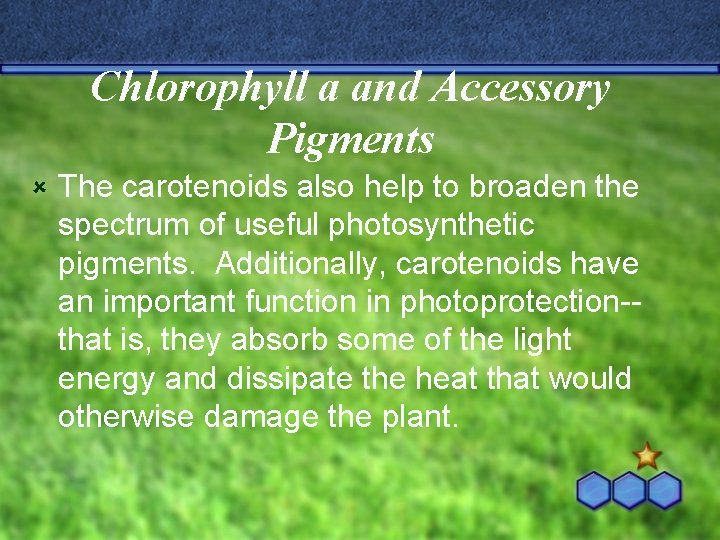
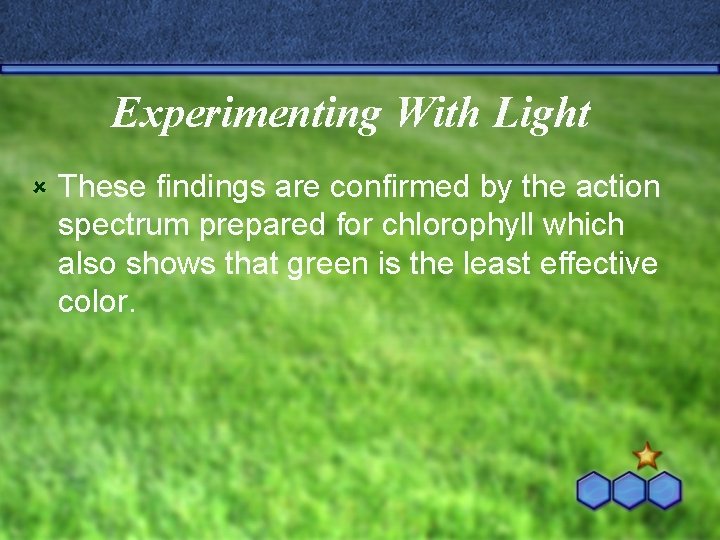
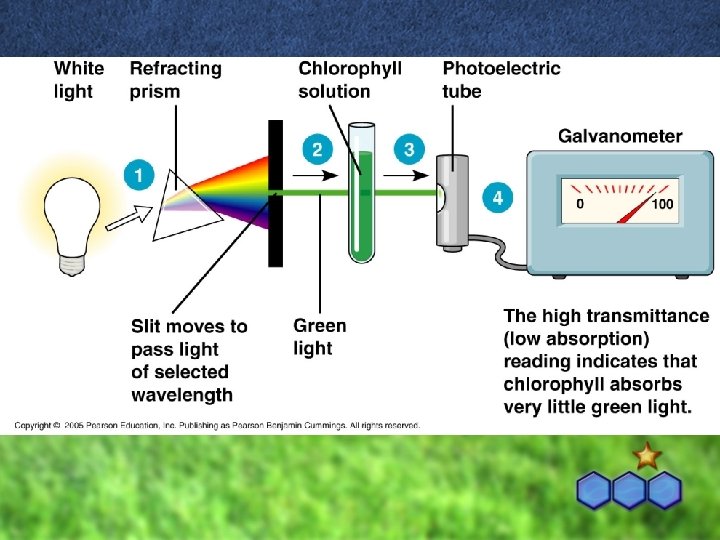
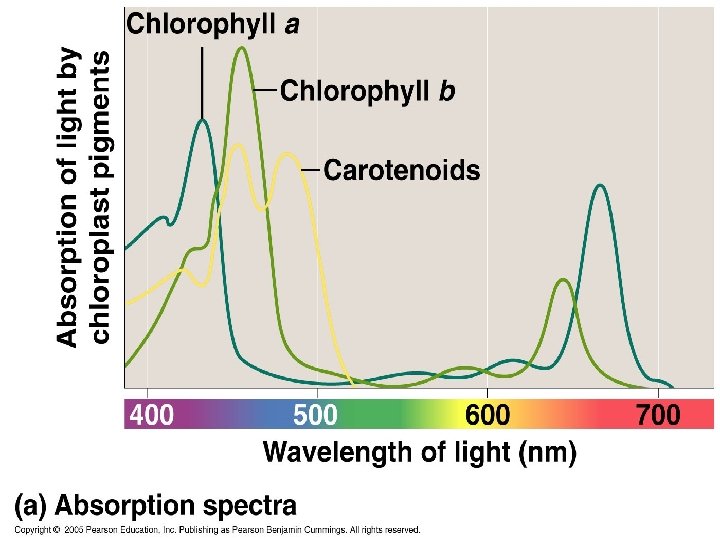
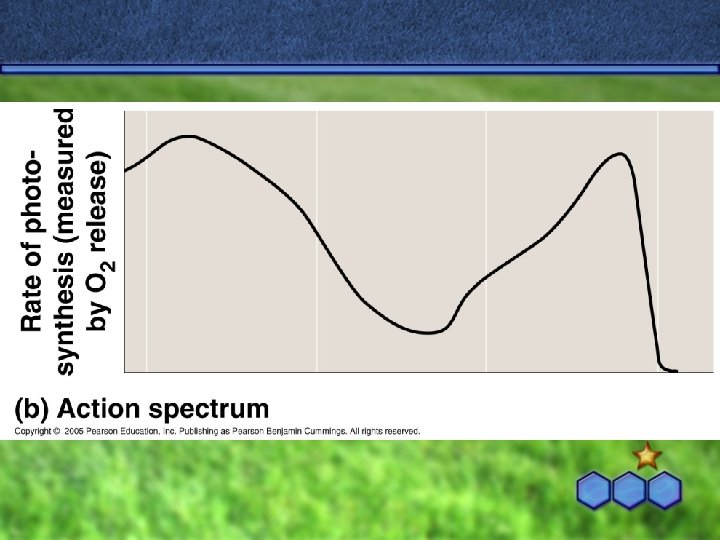
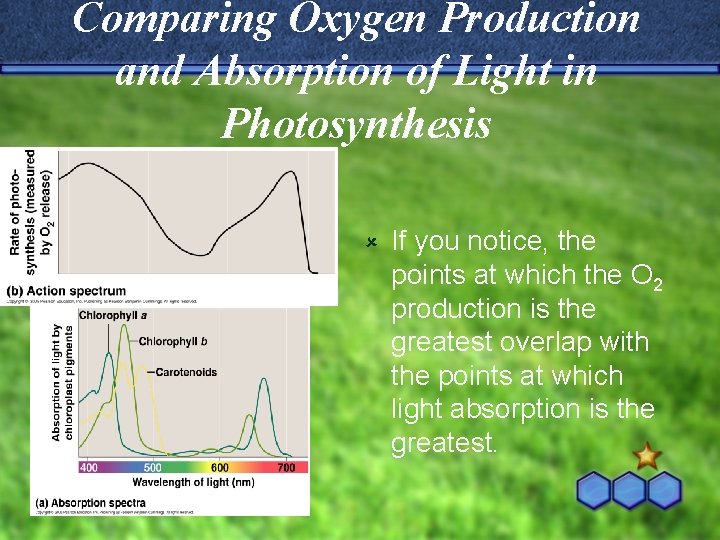
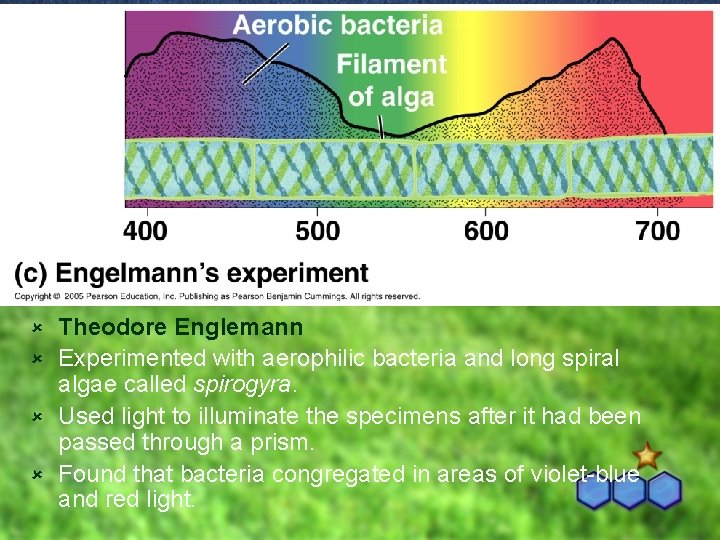
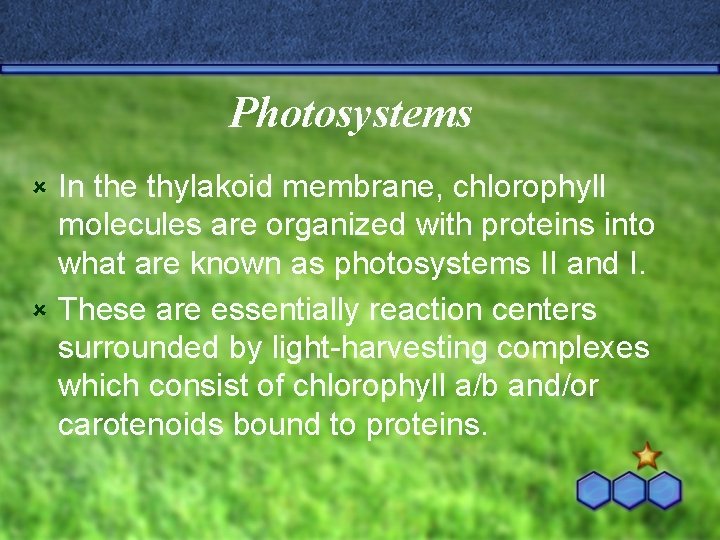
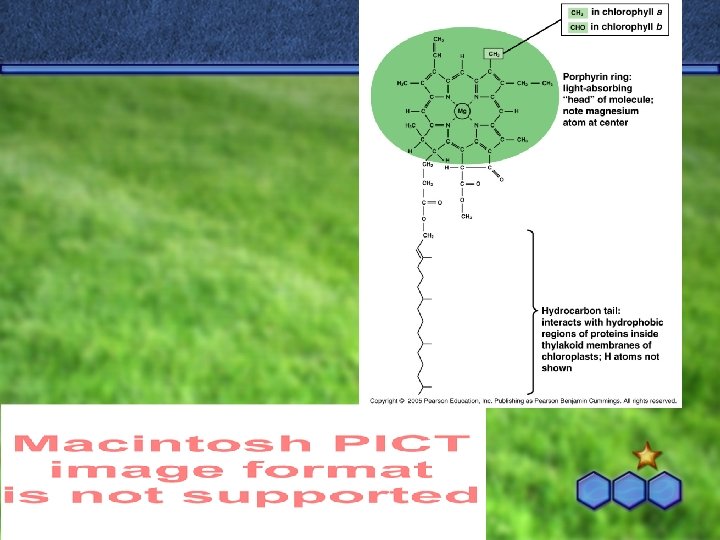
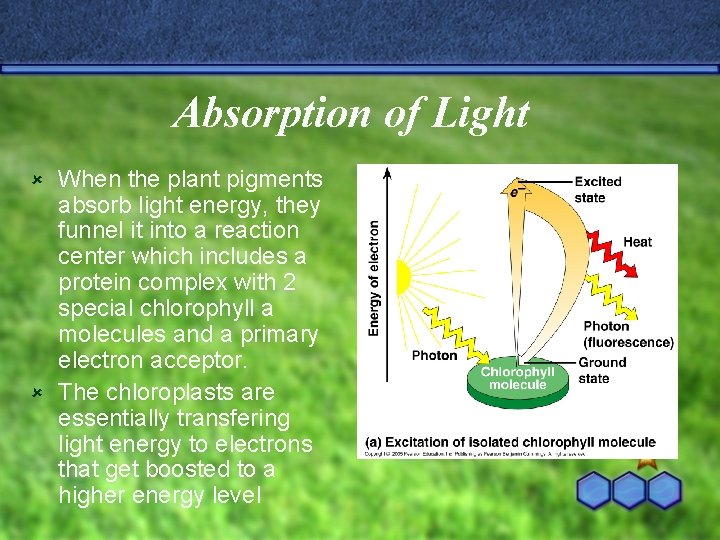
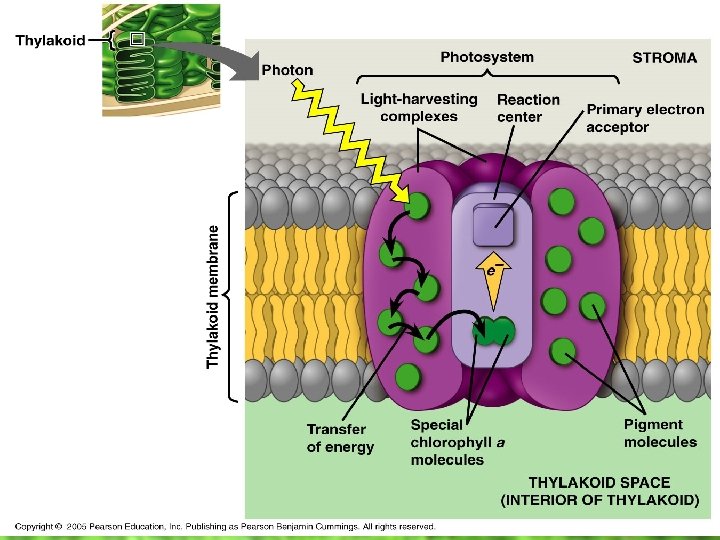
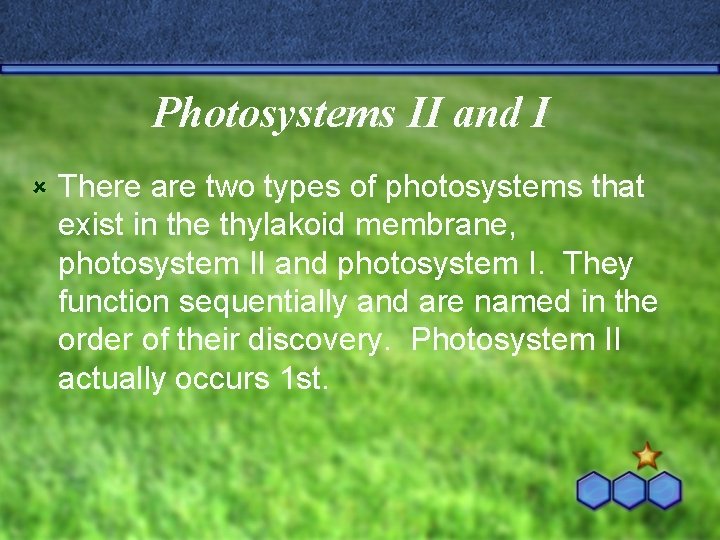
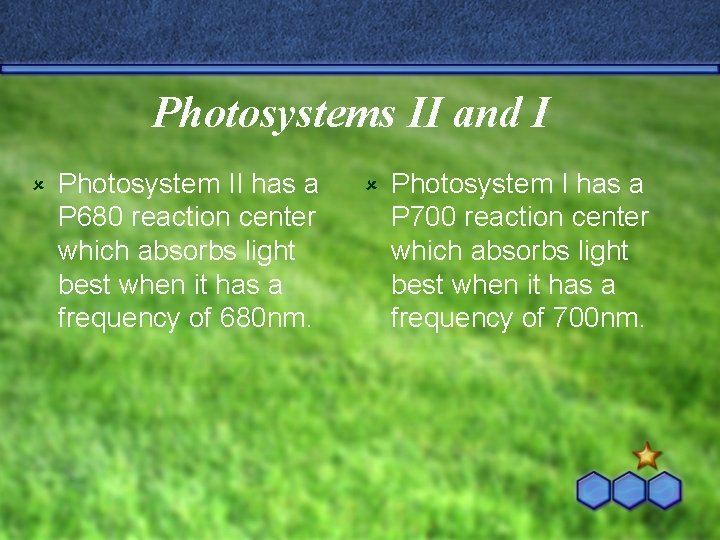
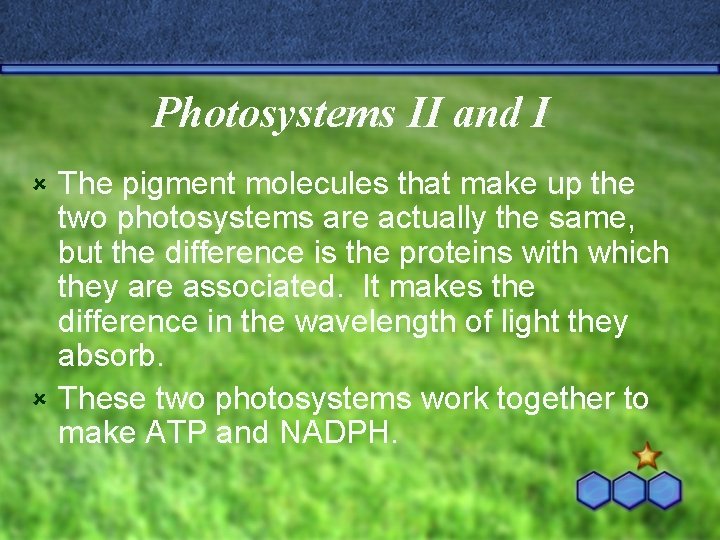
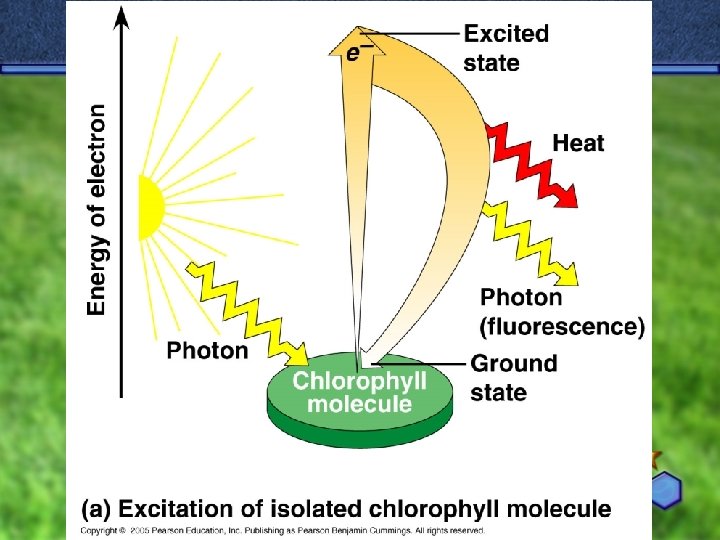
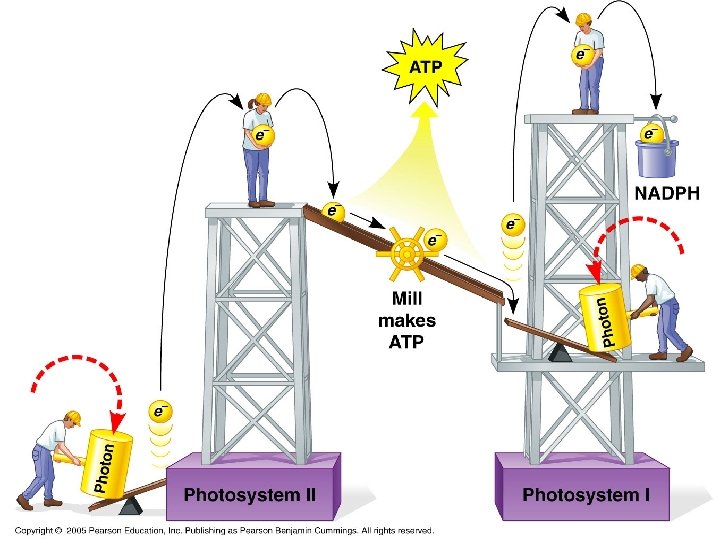
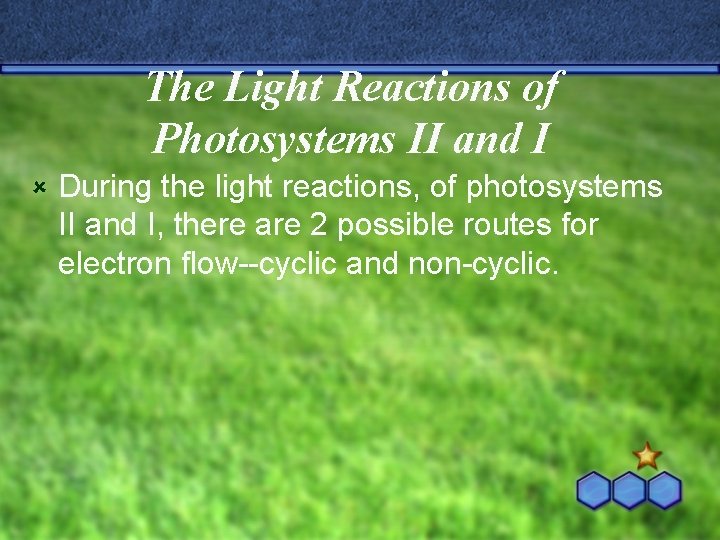
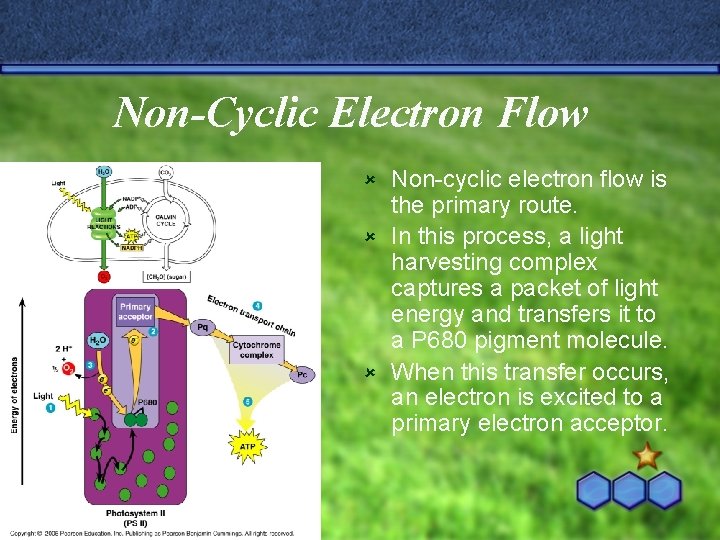
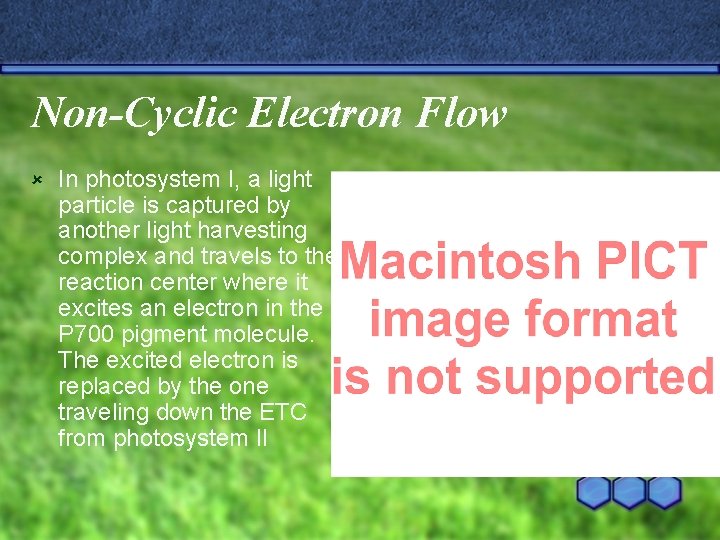
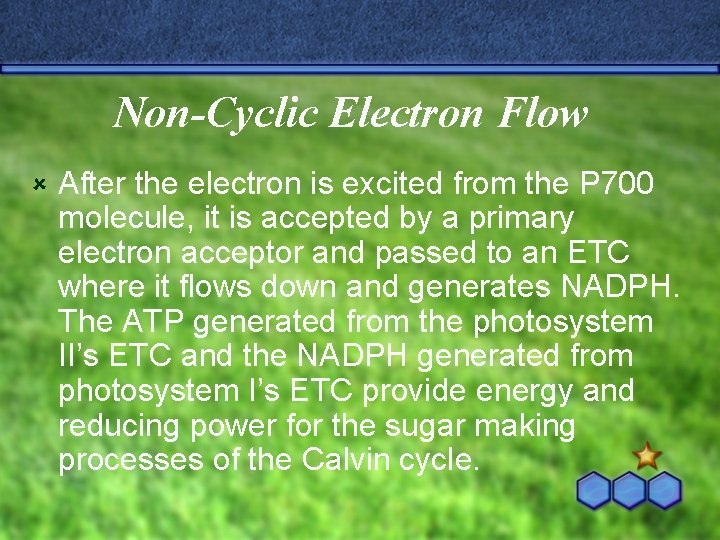
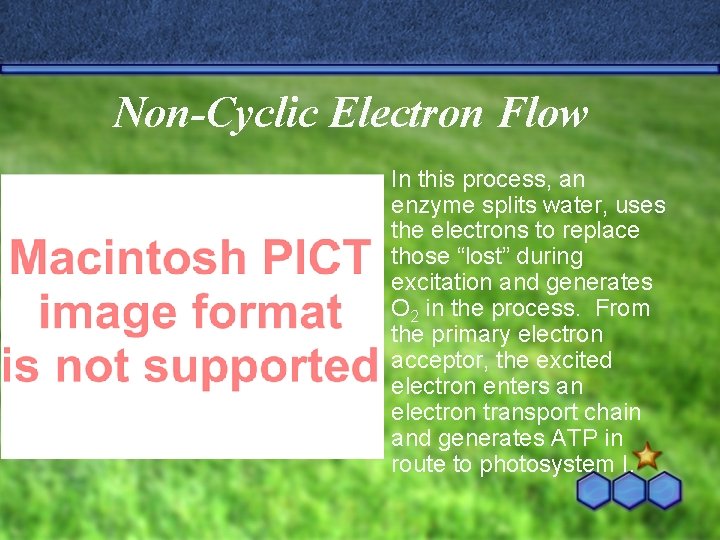
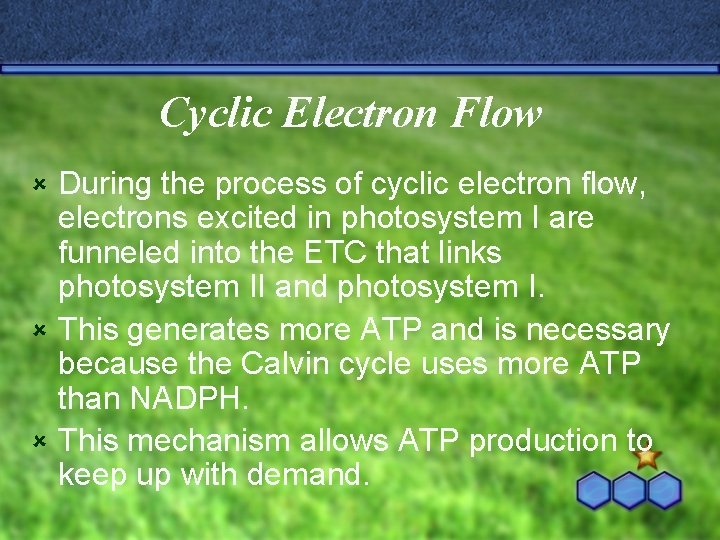
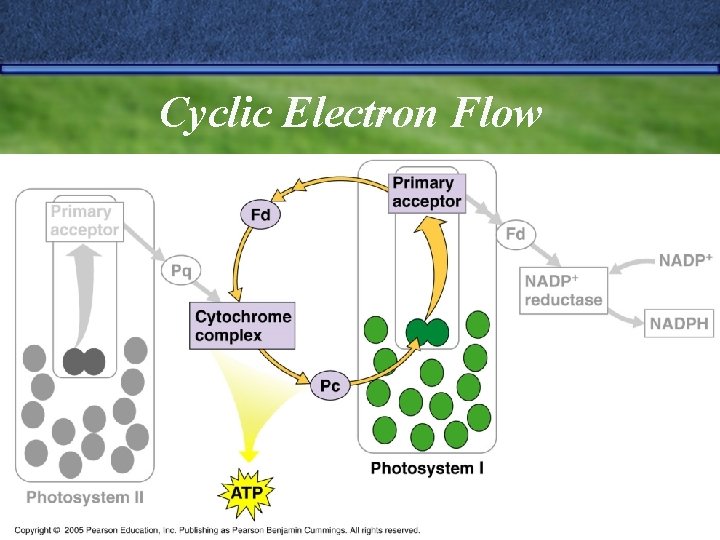
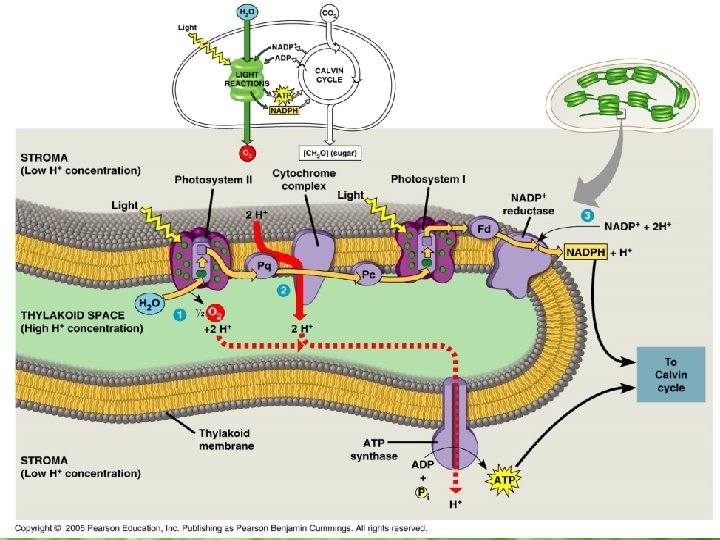
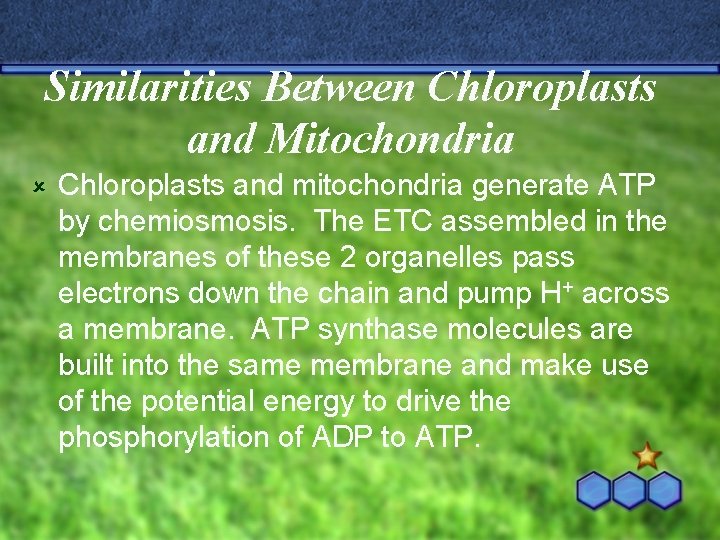
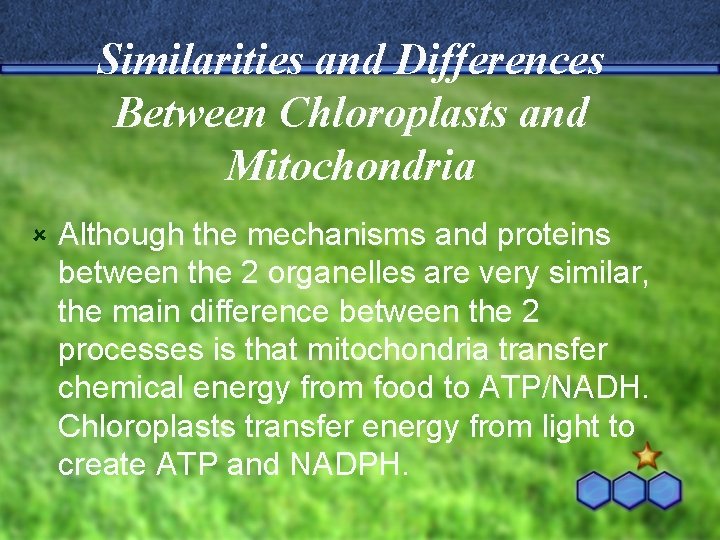
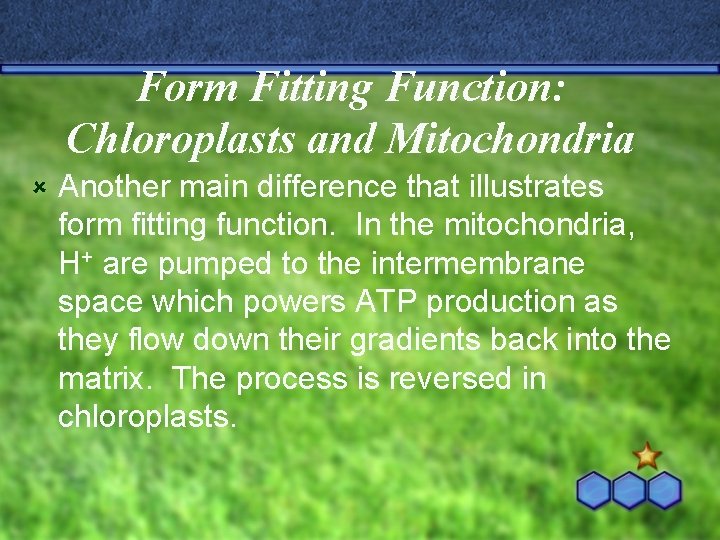
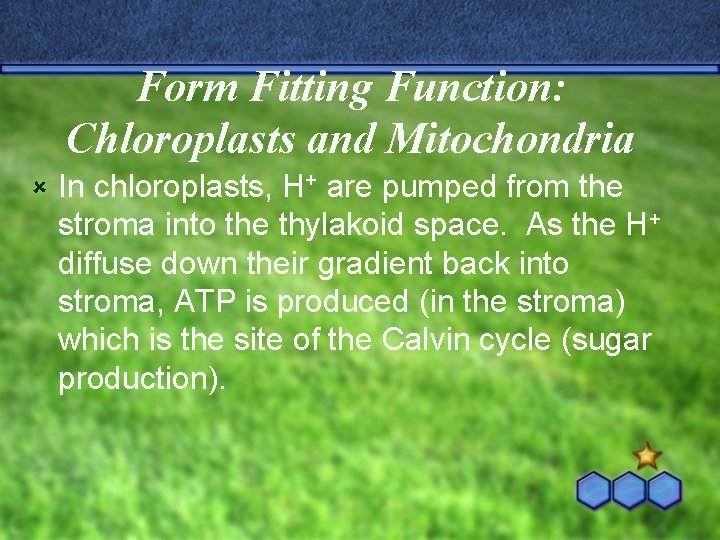
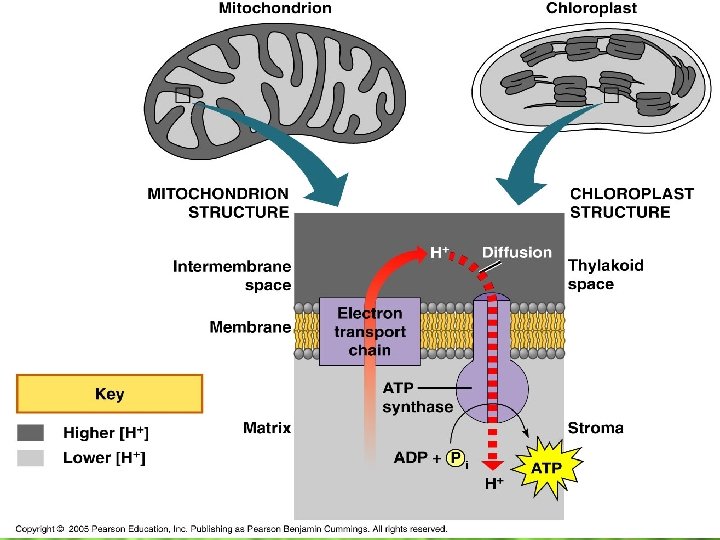
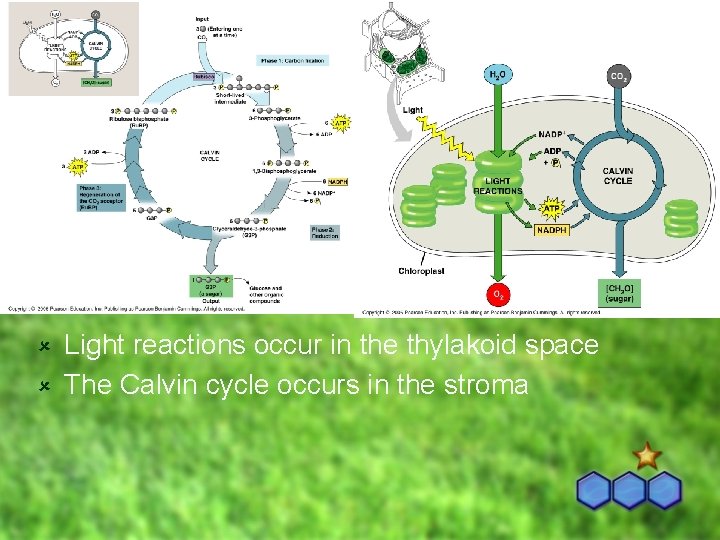
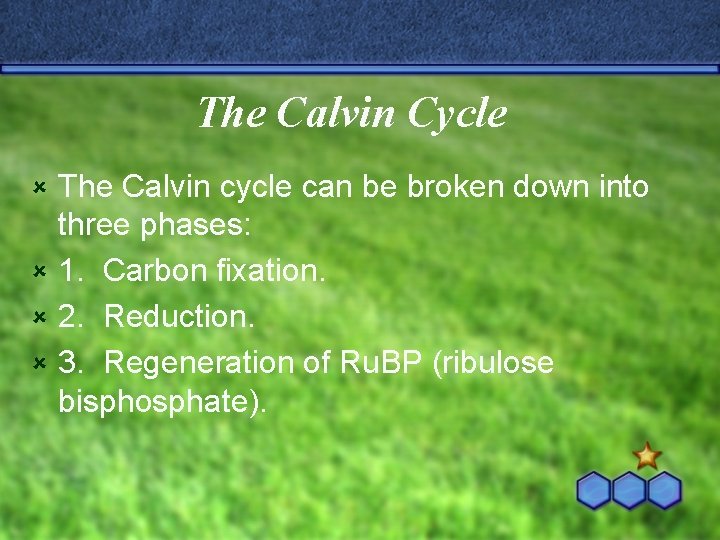
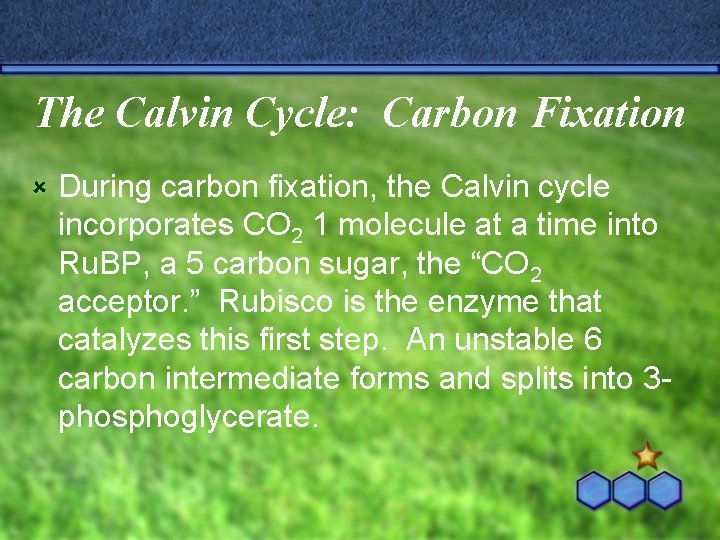
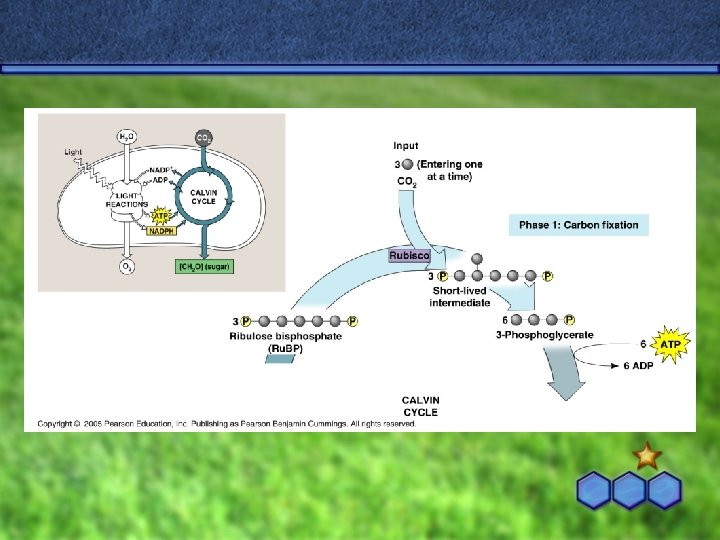
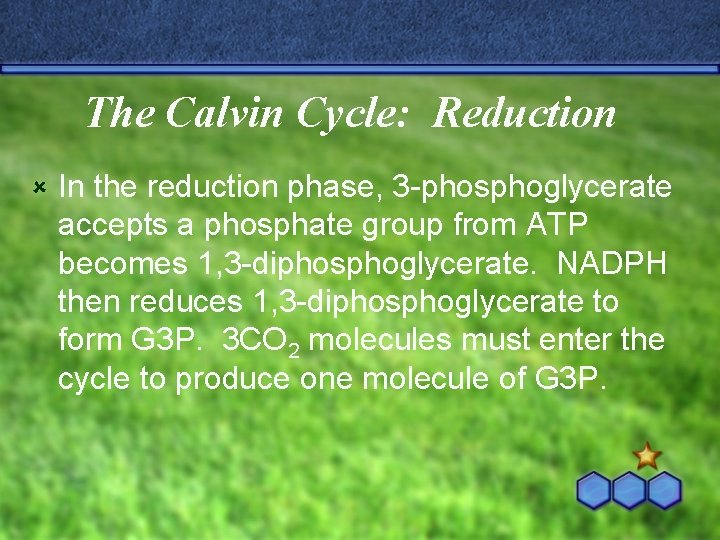
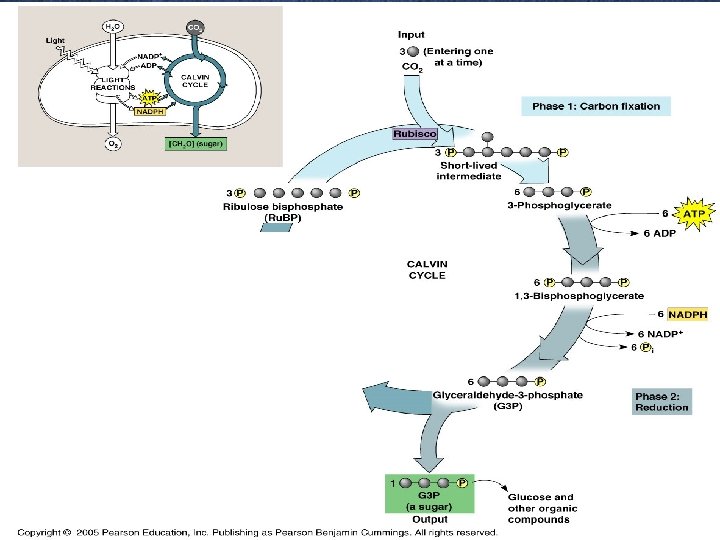
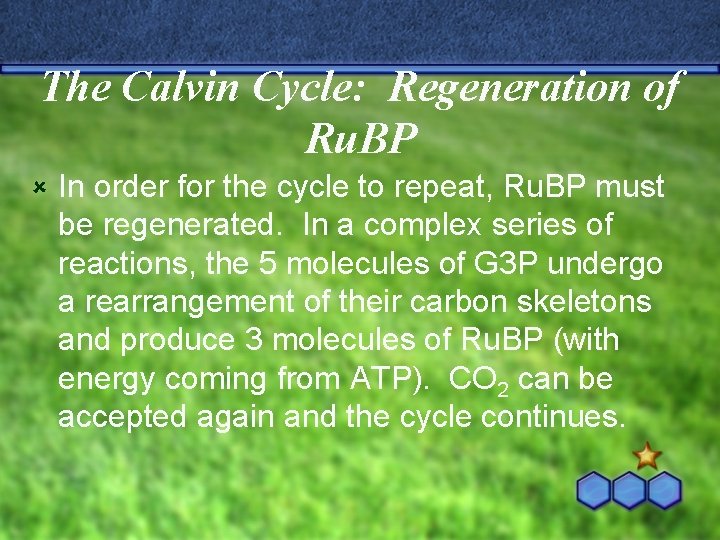
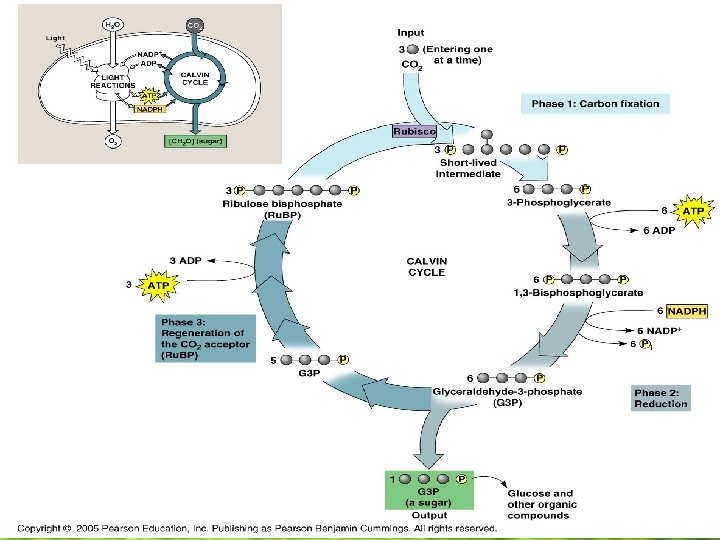
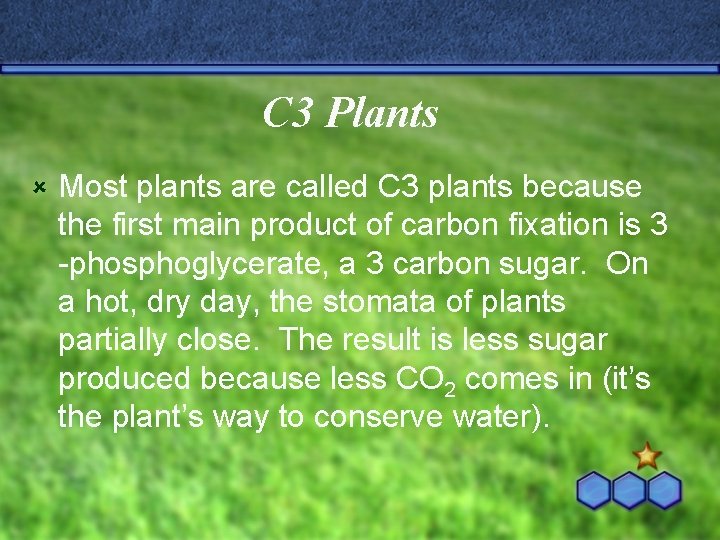
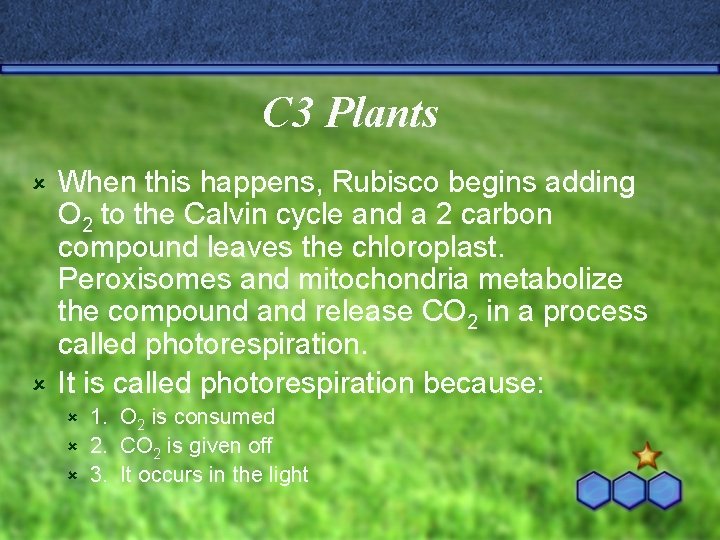
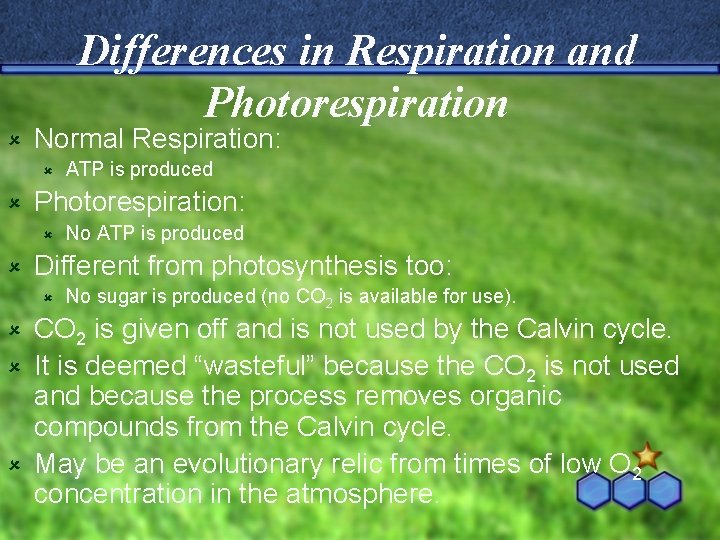
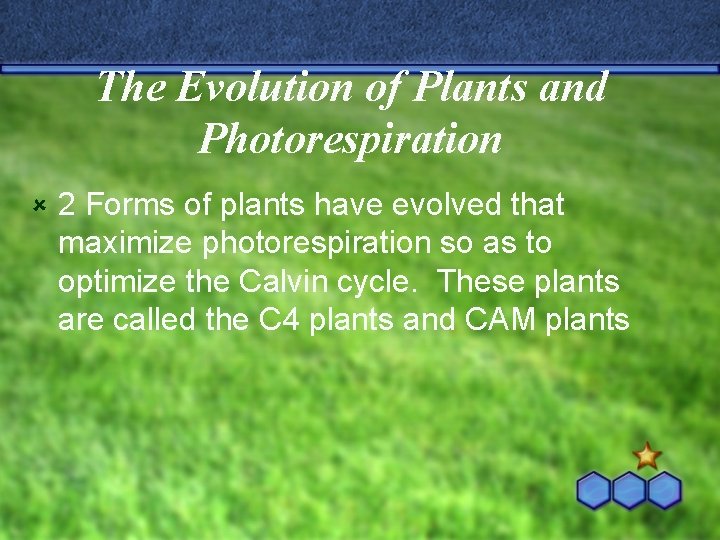
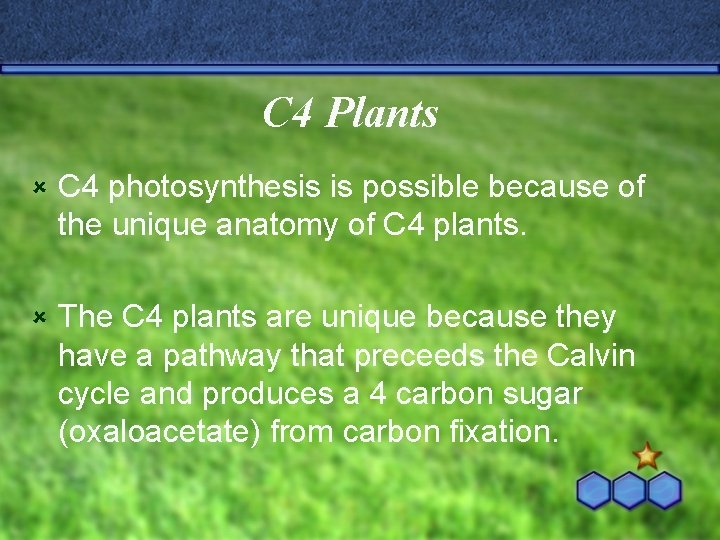
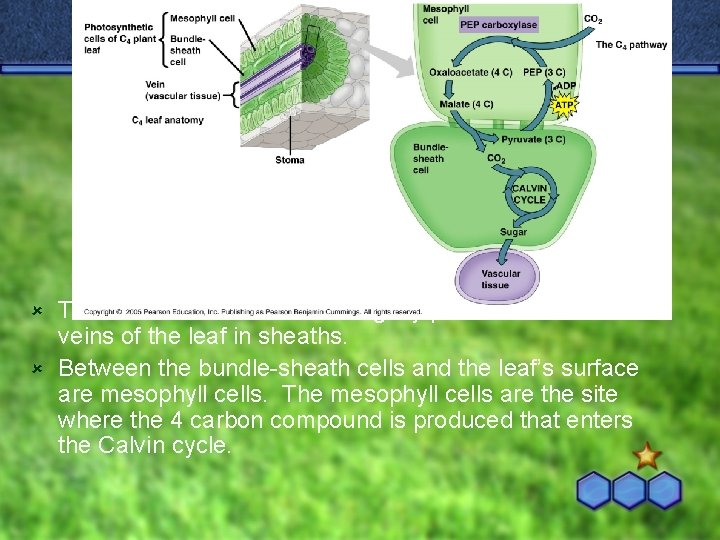
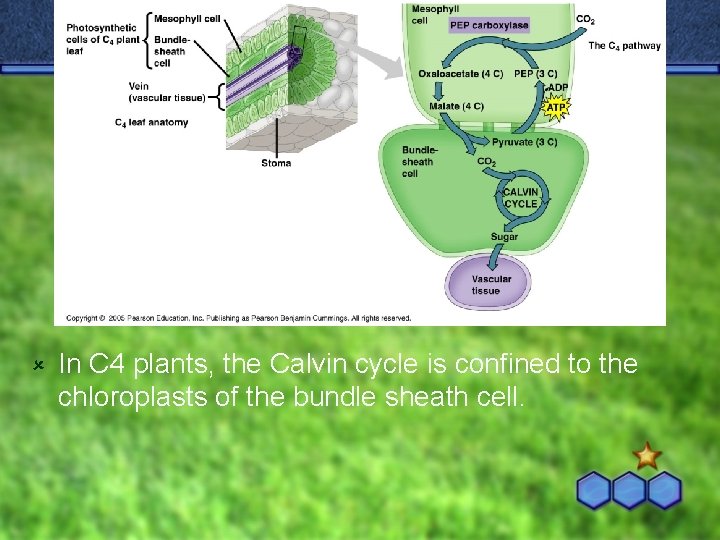
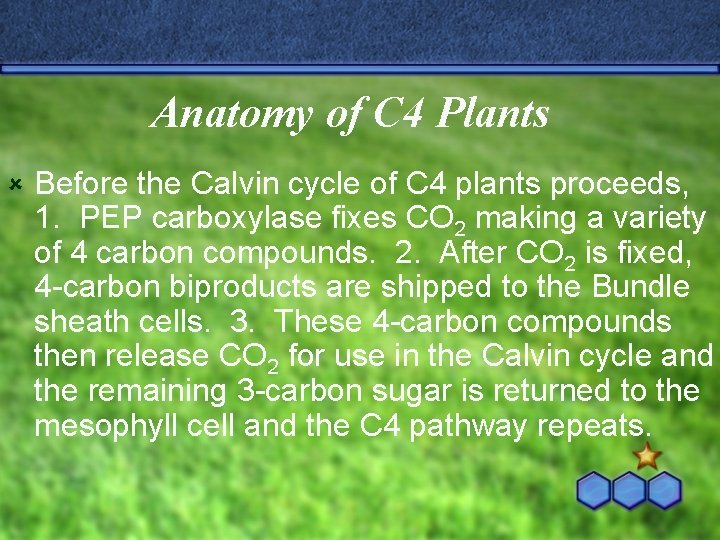
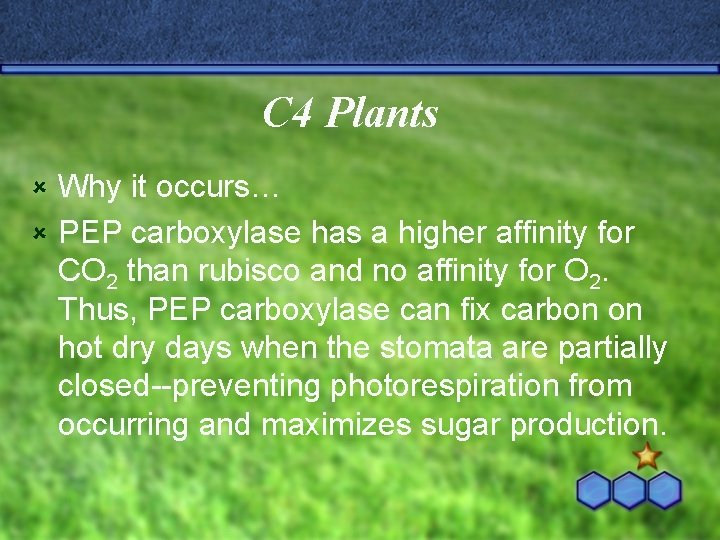
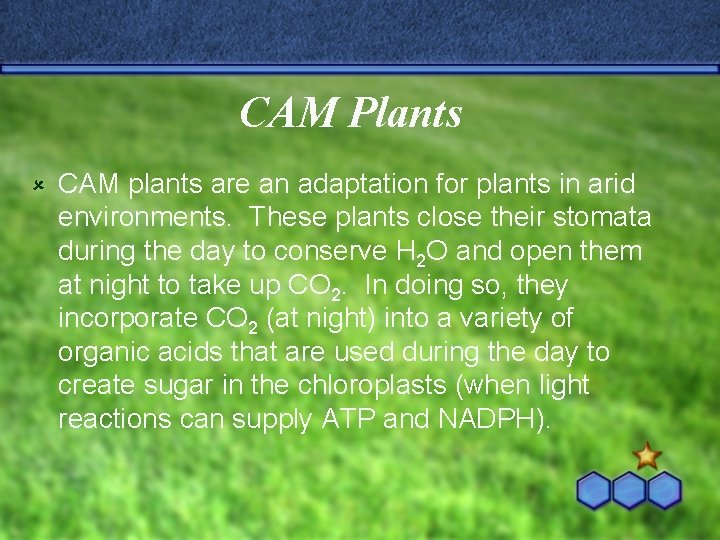
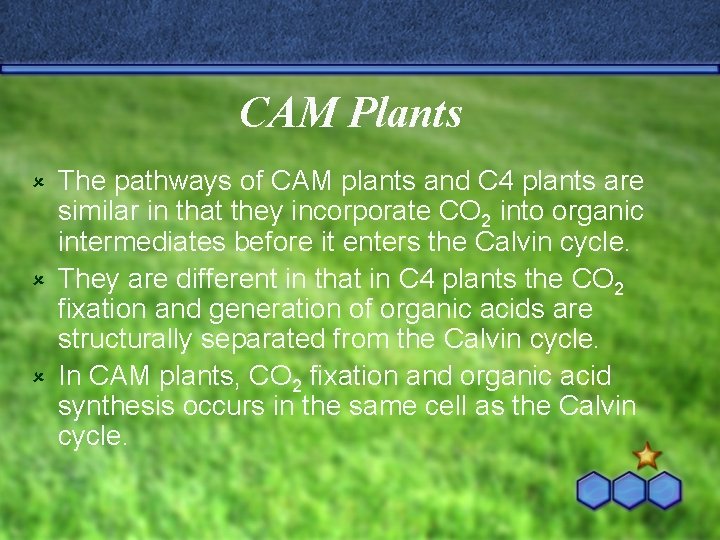
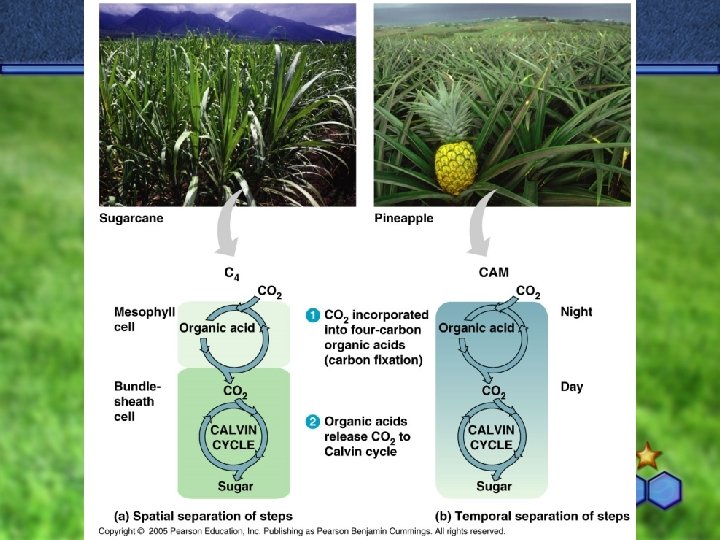
- Slides: 77
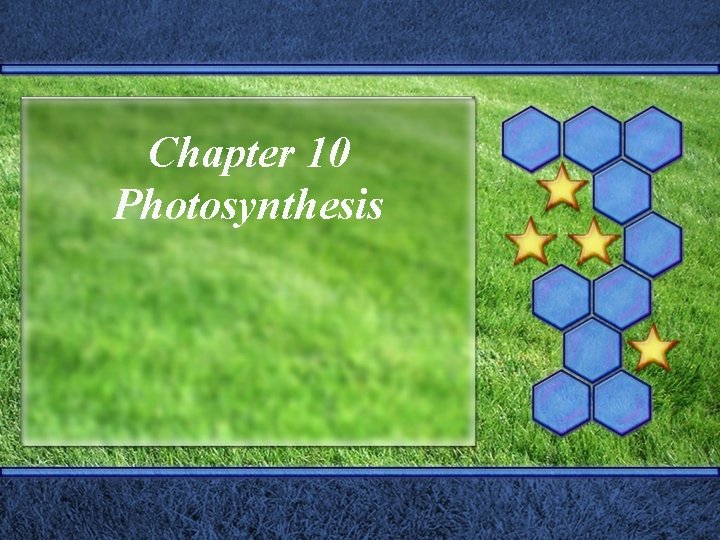
Chapter 10 Photosynthesis
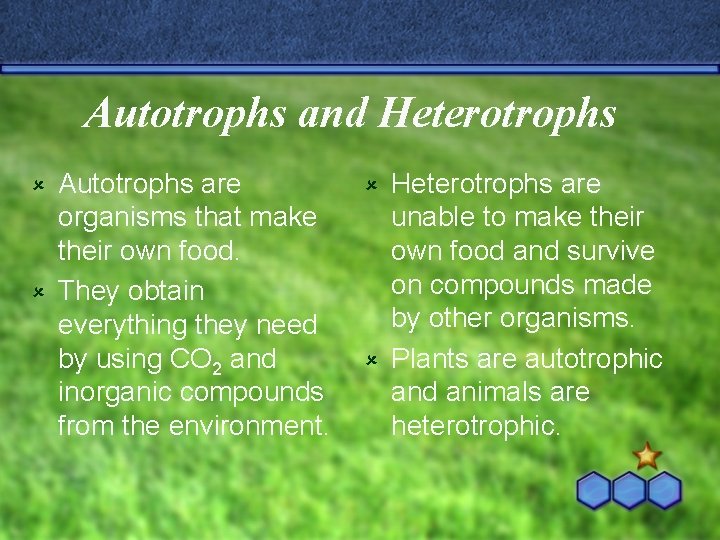
Autotrophs and Heterotrophs Autotrophs are organisms that make their own food. û They obtain everything they need by using CO 2 and inorganic compounds from the environment. û Heterotrophs are unable to make their own food and survive on compounds made by other organisms. û Plants are autotrophic and animals are heterotrophic. û
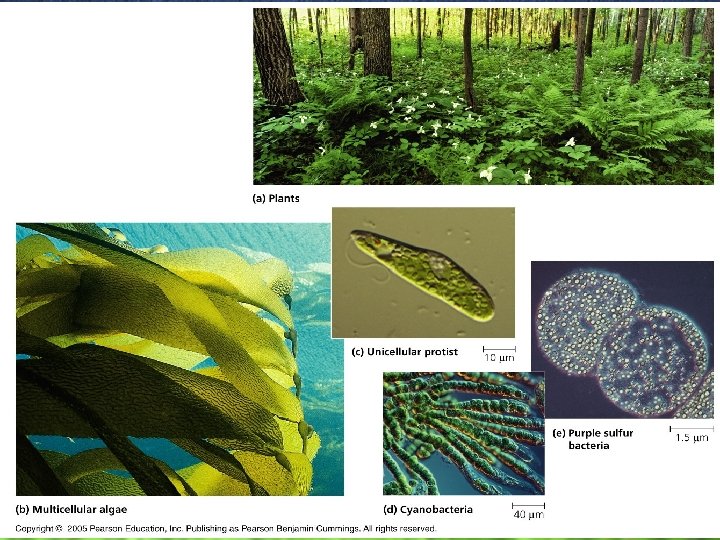
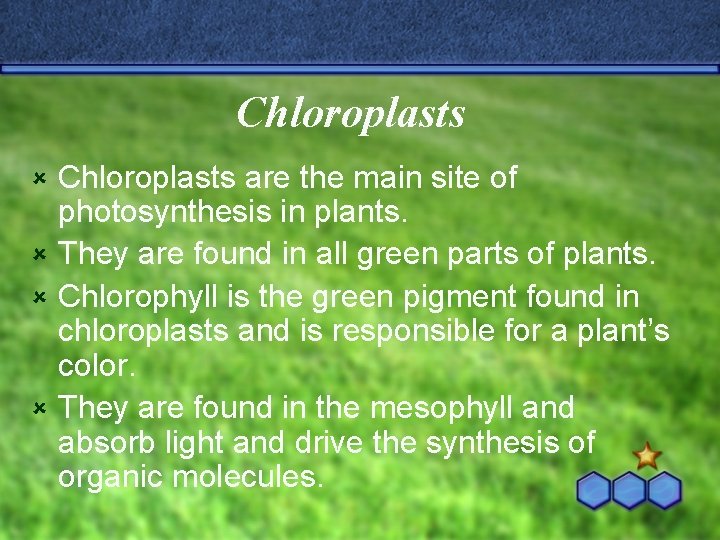
Chloroplasts are the main site of photosynthesis in plants. û They are found in all green parts of plants. û Chlorophyll is the green pigment found in chloroplasts and is responsible for a plant’s color. û They are found in the mesophyll and absorb light and drive the synthesis of organic molecules. û
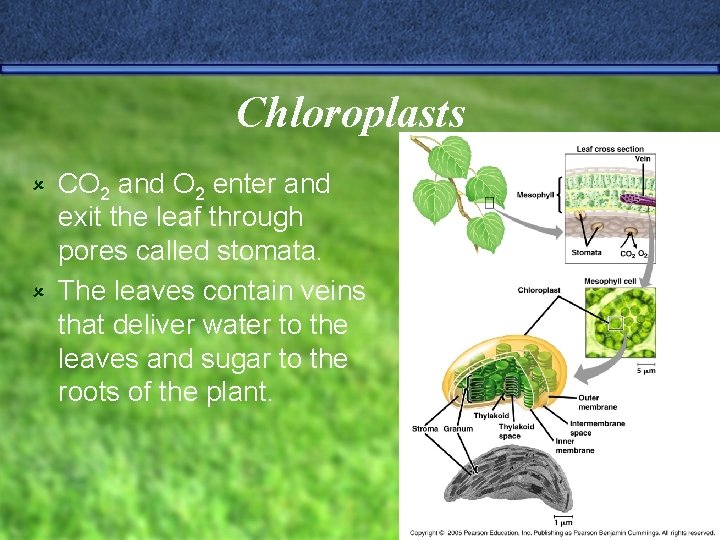
Chloroplasts CO 2 and O 2 enter and exit the leaf through pores called stomata. û The leaves contain veins that deliver water to the leaves and sugar to the roots of the plant. û
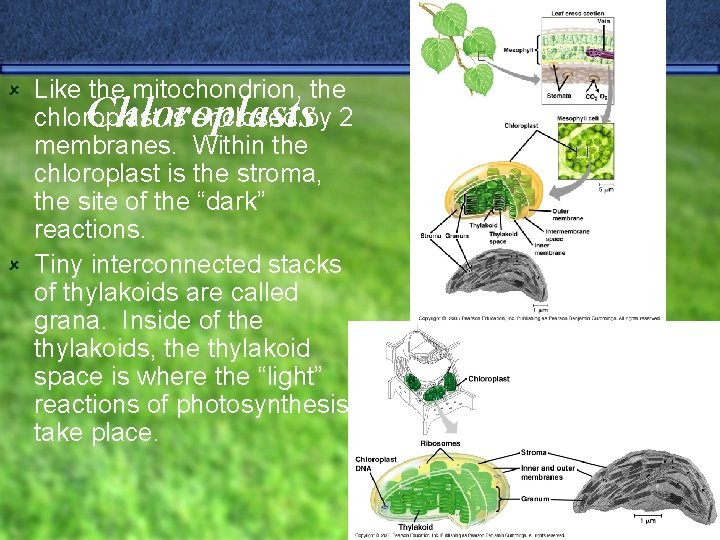
Like the mitochondrion, the chloroplast is enclosed by 2 membranes. Within the chloroplast is the stroma, the site of the “dark” reactions. û Tiny interconnected stacks of thylakoids are called grana. Inside of the thylakoids, the thylakoid space is where the “light” reactions of photosynthesis take place. û Chloroplasts
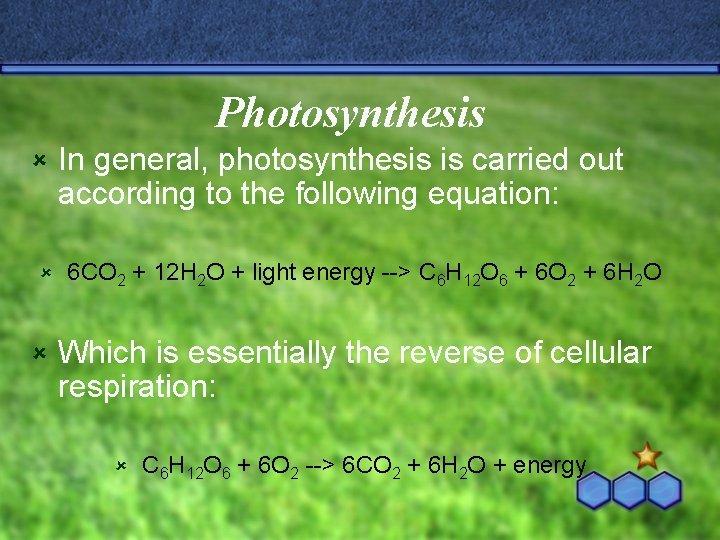
Photosynthesis û û û In general, photosynthesis is carried out according to the following equation: 6 CO 2 + 12 H 2 O + light energy --> C 6 H 12 O 6 + 6 O 2 + 6 H 2 O Which is essentially the reverse of cellular respiration: û C 6 H 12 O 6 + 6 O 2 --> 6 CO 2 + 6 H 2 O + energy
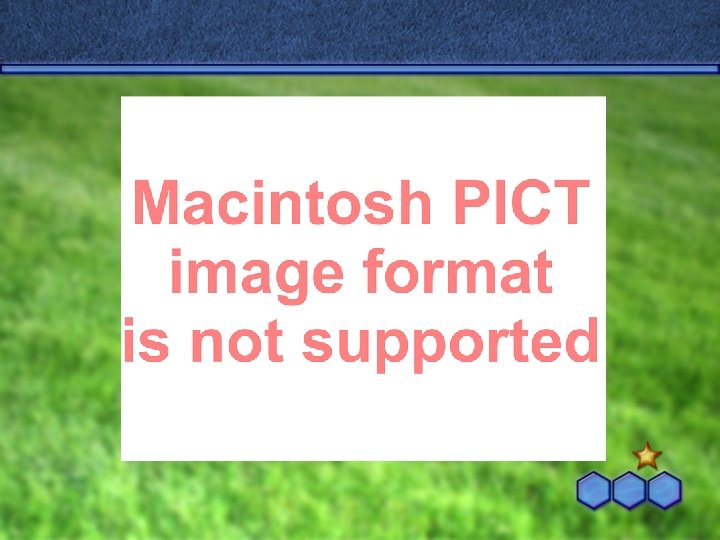
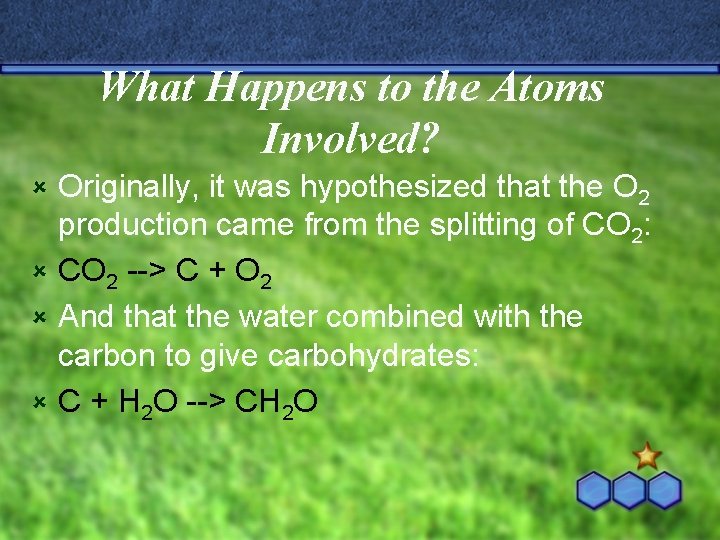
What Happens to the Atoms Involved? Originally, it was hypothesized that the O 2 production came from the splitting of CO 2: û CO 2 --> C + O 2 û And that the water combined with the carbon to give carbohydrates: û C + H 2 O --> CH 2 O û
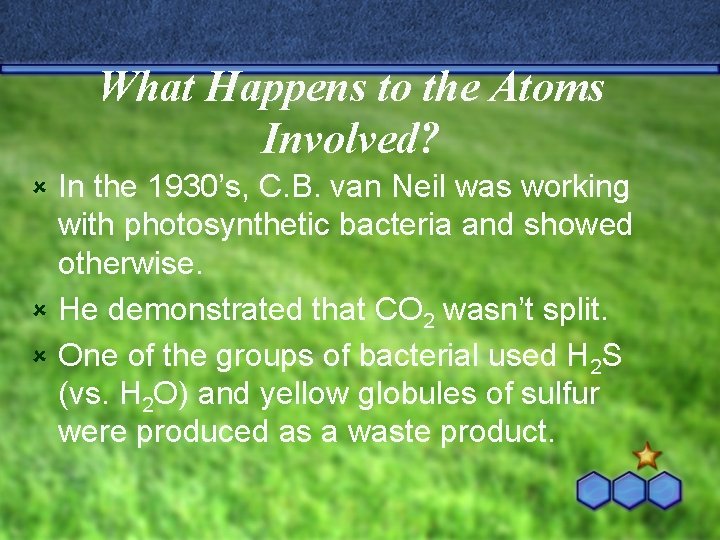
What Happens to the Atoms Involved? In the 1930’s, C. B. van Neil was working with photosynthetic bacteria and showed otherwise. û He demonstrated that CO 2 wasn’t split. û One of the groups of bacterial used H 2 S (vs. H 2 O) and yellow globules of sulfur were produced as a waste product. û
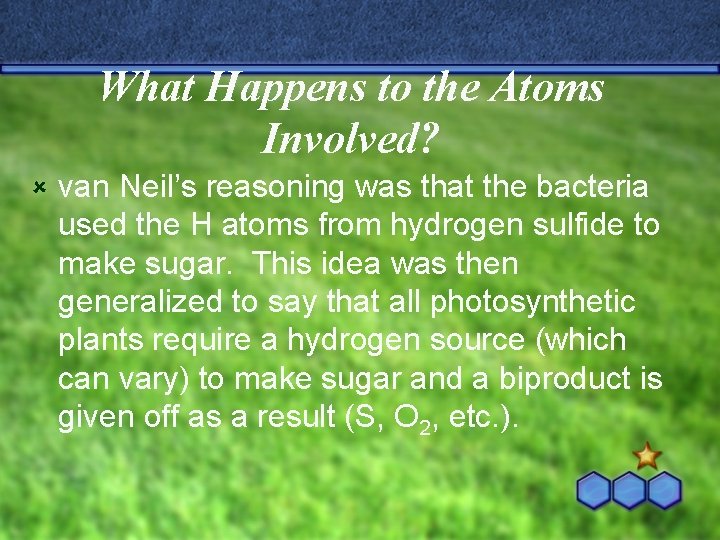
What Happens to the Atoms Involved? û van Neil’s reasoning was that the bacteria used the H atoms from hydrogen sulfide to make sugar. This idea was then generalized to say that all photosynthetic plants require a hydrogen source (which can vary) to make sugar and a biproduct is given off as a result (S, O 2, etc. ).
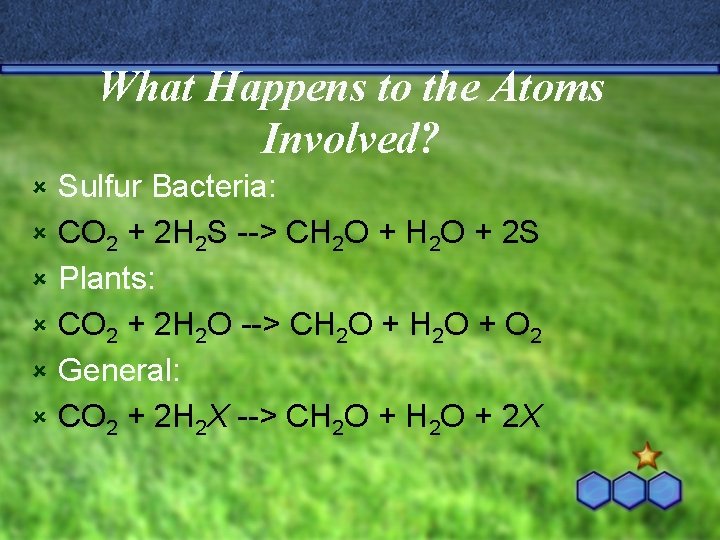
What Happens to the Atoms Involved? û û û Sulfur Bacteria: CO 2 + 2 H 2 S --> CH 2 O + 2 S Plants: CO 2 + 2 H 2 O --> CH 2 O + O 2 General: CO 2 + 2 H 2 X --> CH 2 O + 2 X
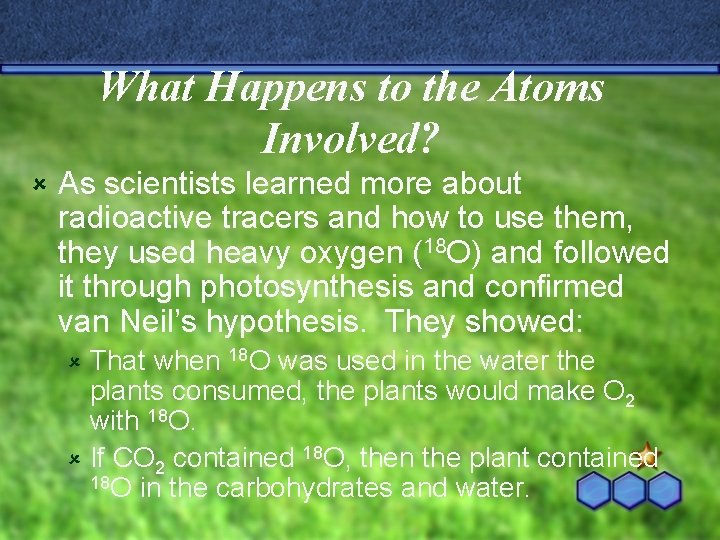
What Happens to the Atoms Involved? û As scientists learned more about radioactive tracers and how to use them, they used heavy oxygen (18 O) and followed it through photosynthesis and confirmed van Neil’s hypothesis. They showed: That when 18 O was used in the water the plants consumed, the plants would make O 2 with 18 O. û If CO 2 contained 18 O, then the plant contained 18 O in the carbohydrates and water. û
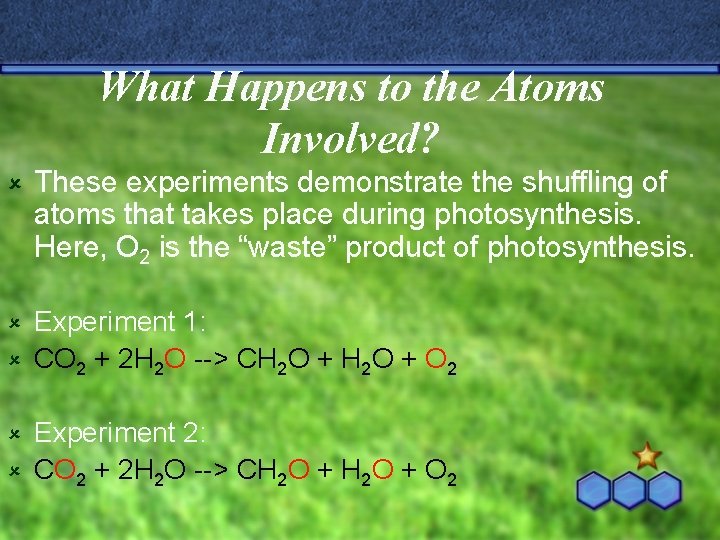
What Happens to the Atoms Involved? û These experiments demonstrate the shuffling of atoms that takes place during photosynthesis. Here, O 2 is the “waste” product of photosynthesis. Experiment 1: û CO 2 + 2 H 2 O --> CH 2 O + O 2 û Experiment 2: û CO 2 + 2 H 2 O --> CH 2 O + O 2 û
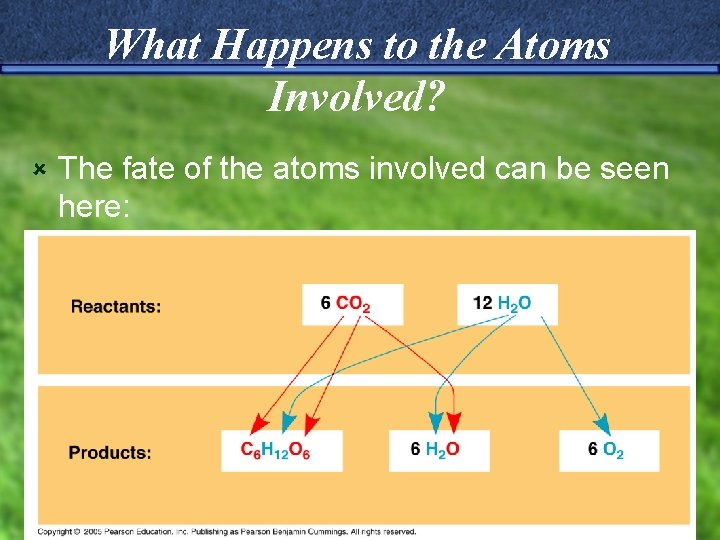
What Happens to the Atoms Involved? û The fate of the atoms involved can be seen here:
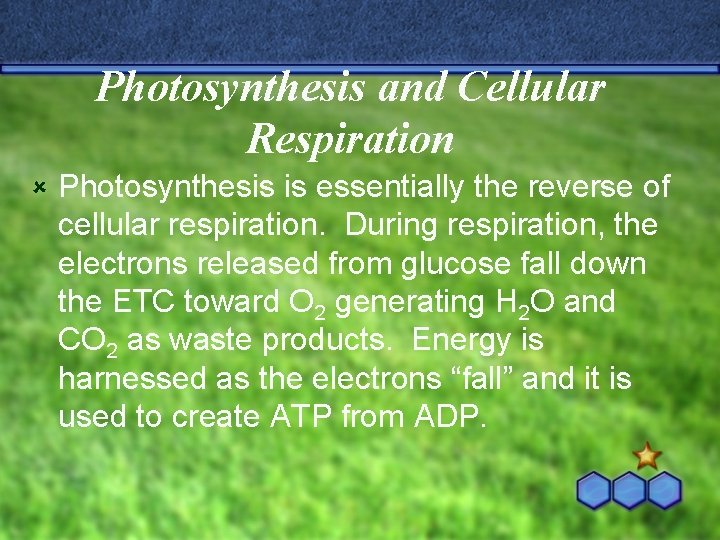
Photosynthesis and Cellular Respiration û Photosynthesis is essentially the reverse of cellular respiration. During respiration, the electrons released from glucose fall down the ETC toward O 2 generating H 2 O and CO 2 as waste products. Energy is harnessed as the electrons “fall” and it is used to create ATP from ADP.
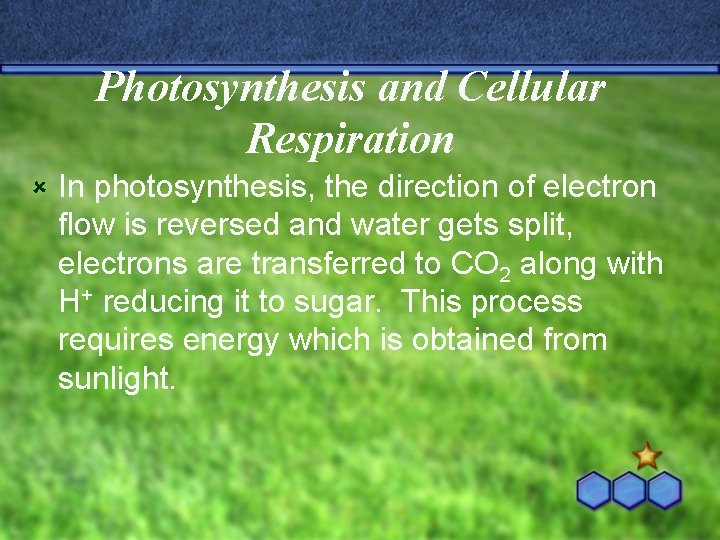
Photosynthesis and Cellular Respiration û In photosynthesis, the direction of electron flow is reversed and water gets split, electrons are transferred to CO 2 along with H+ reducing it to sugar. This process requires energy which is obtained from sunlight.
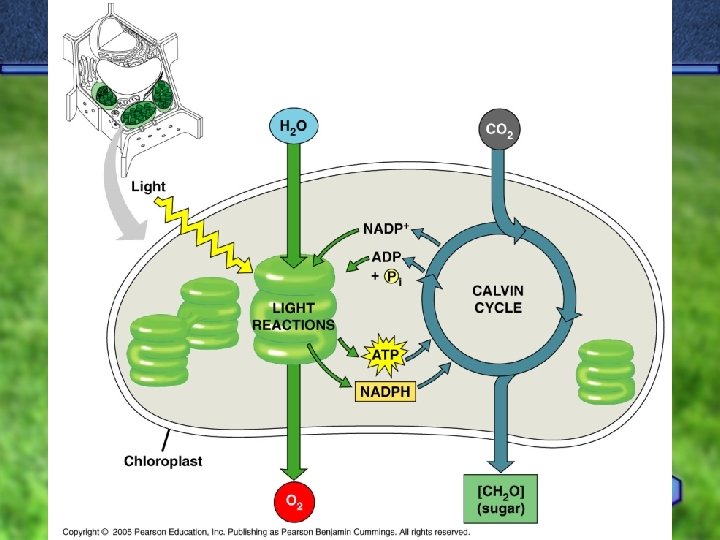
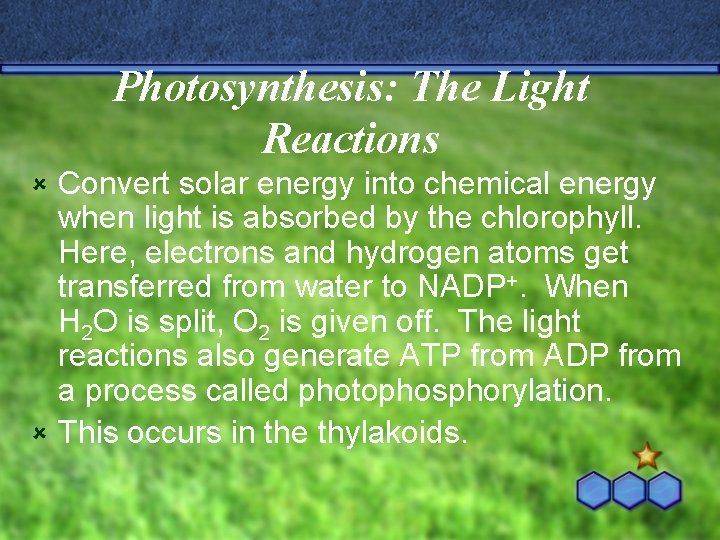
Photosynthesis: The Light Reactions Convert solar energy into chemical energy when light is absorbed by the chlorophyll. Here, electrons and hydrogen atoms get transferred from water to NADP+. When H 2 O is split, O 2 is given off. The light reactions also generate ATP from ADP from a process called photophosphorylation. û This occurs in the thylakoids. û
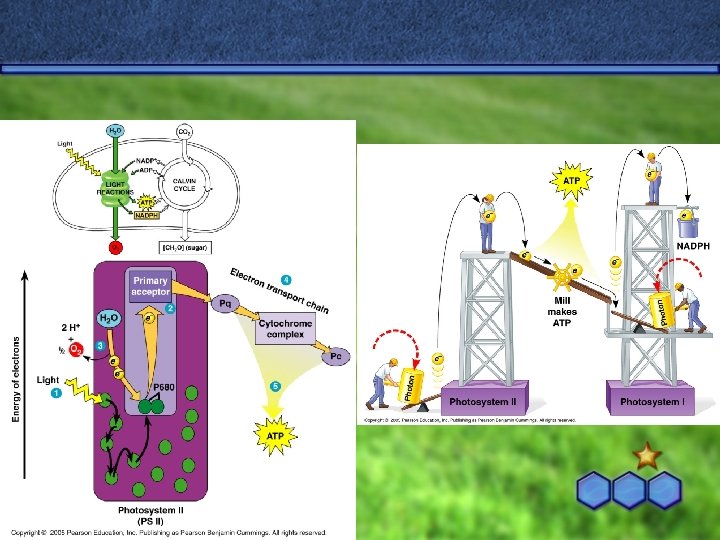
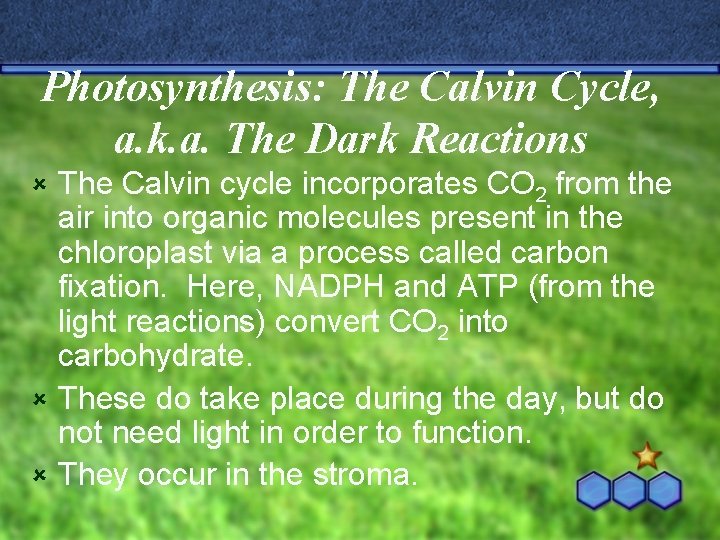
Photosynthesis: The Calvin Cycle, a. k. a. The Dark Reactions The Calvin cycle incorporates CO 2 from the air into organic molecules present in the chloroplast via a process called carbon fixation. Here, NADPH and ATP (from the light reactions) convert CO 2 into carbohydrate. û These do take place during the day, but do not need light in order to function. û They occur in the stroma. û
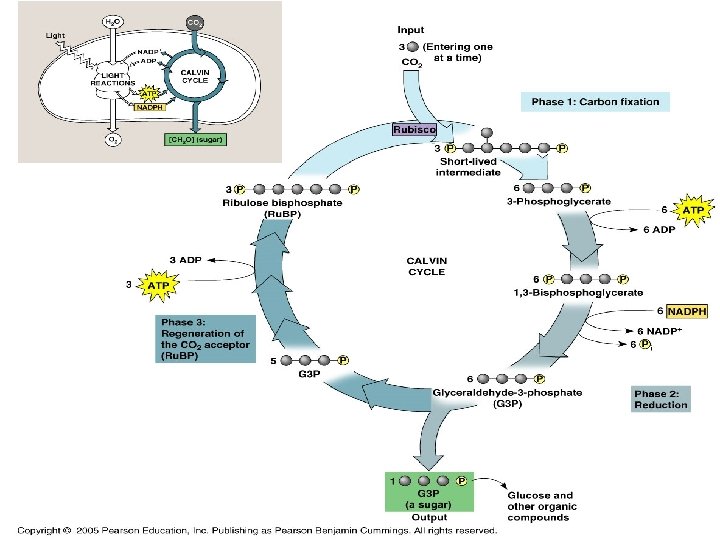
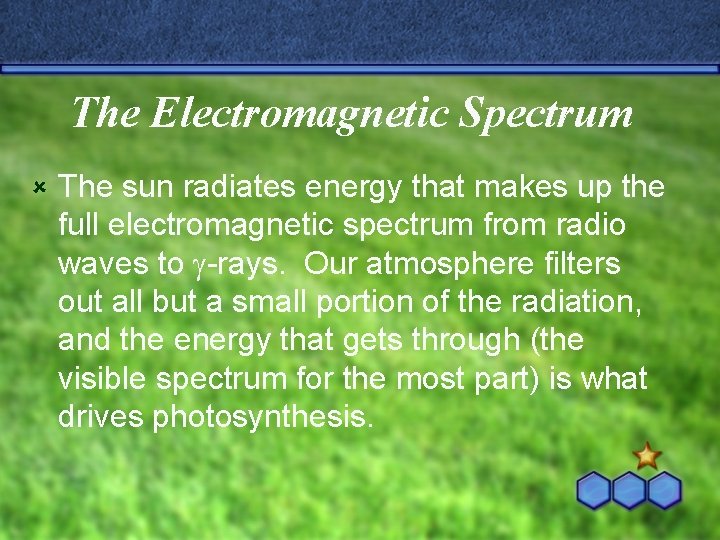
The Electromagnetic Spectrum û The sun radiates energy that makes up the full electromagnetic spectrum from radio waves to -rays. Our atmosphere filters out all but a small portion of the radiation, and the energy that gets through (the visible spectrum for the most part) is what drives photosynthesis.
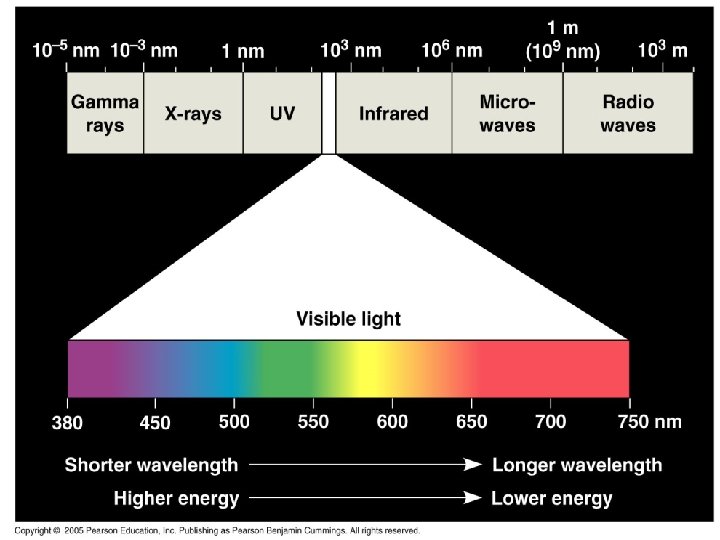
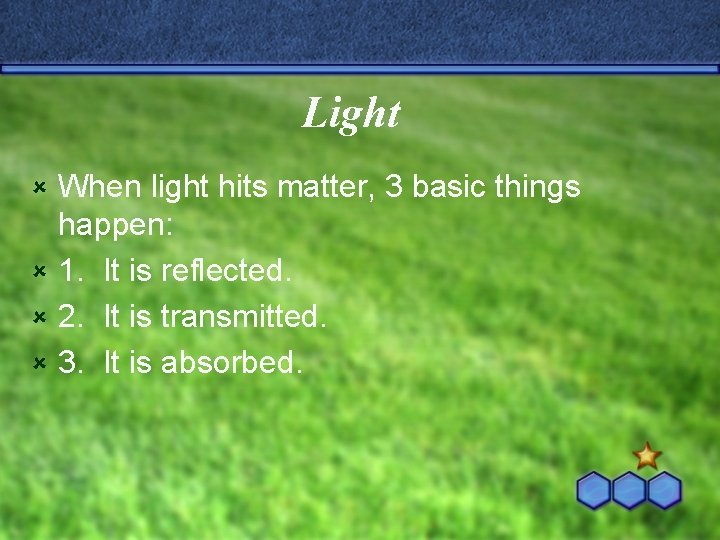
Light When light hits matter, 3 basic things happen: û 1. It is reflected. û 2. It is transmitted. û 3. It is absorbed. û
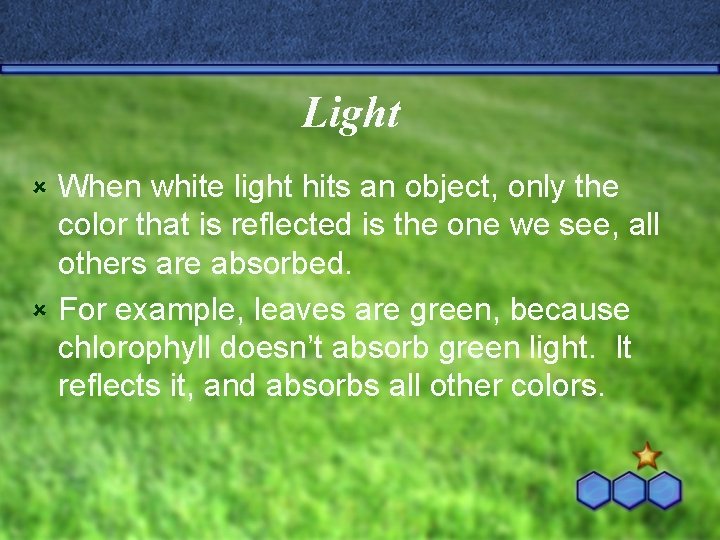
Light When white light hits an object, only the color that is reflected is the one we see, all others are absorbed. û For example, leaves are green, because chlorophyll doesn’t absorb green light. It reflects it, and absorbs all other colors. û
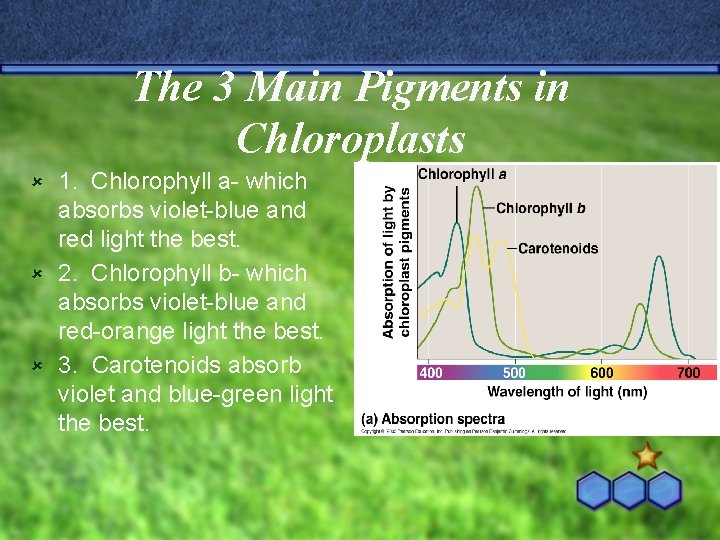
The 3 Main Pigments in Chloroplasts 1. Chlorophyll a- which absorbs violet-blue and red light the best. û 2. Chlorophyll b- which absorbs violet-blue and red-orange light the best. û 3. Carotenoids absorb violet and blue-green light the best. û
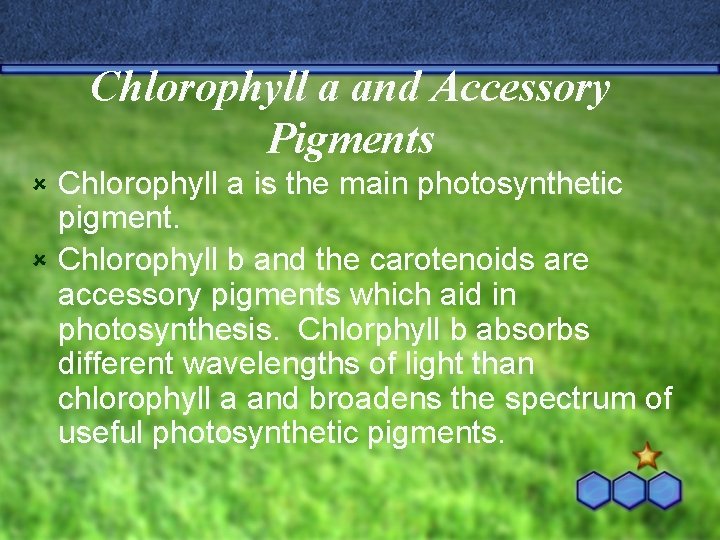
Chlorophyll a and Accessory Pigments Chlorophyll a is the main photosynthetic pigment. û Chlorophyll b and the carotenoids are accessory pigments which aid in photosynthesis. Chlorphyll b absorbs different wavelengths of light than chlorophyll a and broadens the spectrum of useful photosynthetic pigments. û
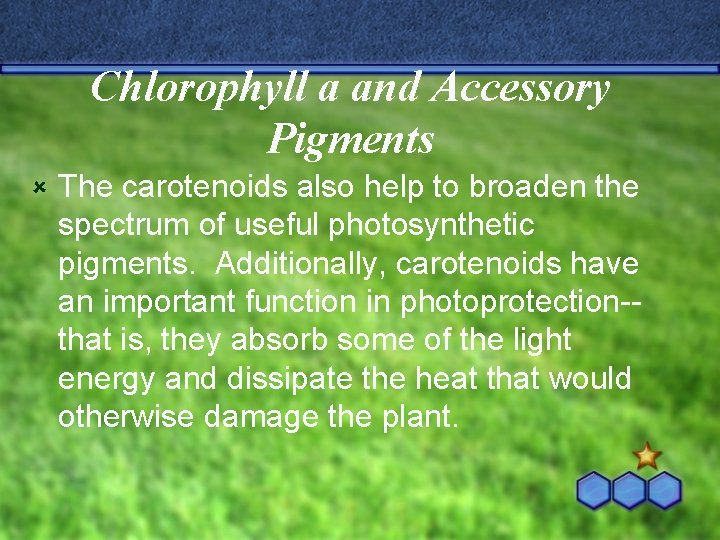
Chlorophyll a and Accessory Pigments û The carotenoids also help to broaden the spectrum of useful photosynthetic pigments. Additionally, carotenoids have an important function in photoprotection-that is, they absorb some of the light energy and dissipate the heat that would otherwise damage the plant.
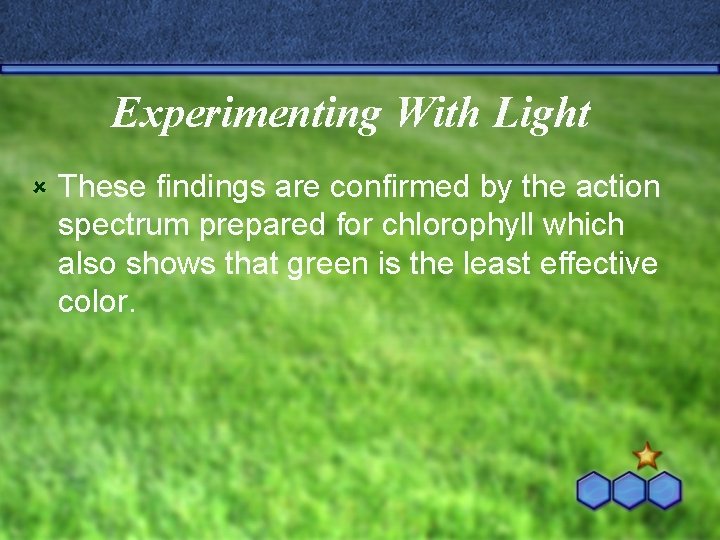
Experimenting With Light û These findings are confirmed by the action spectrum prepared for chlorophyll which also shows that green is the least effective color.
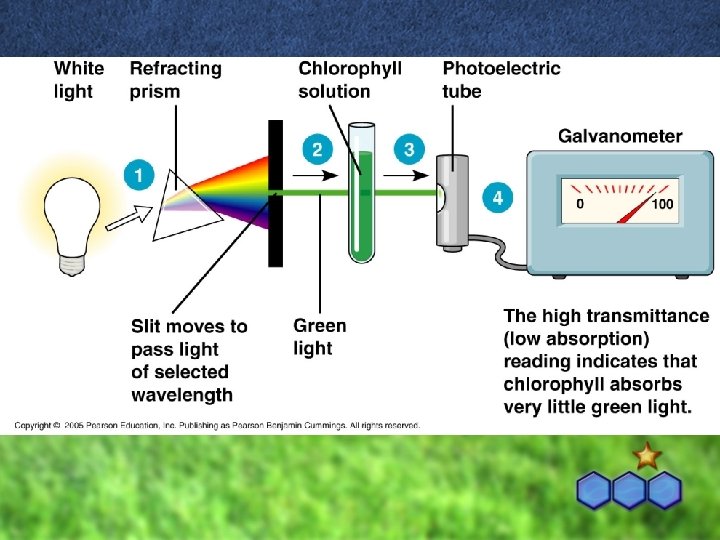
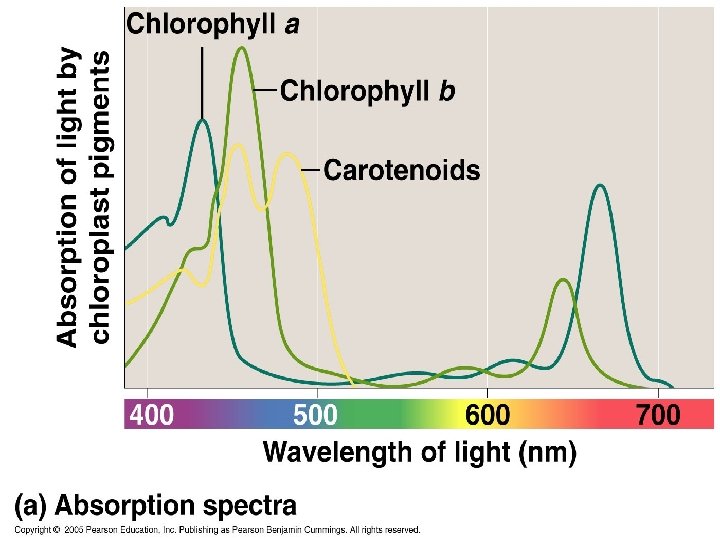
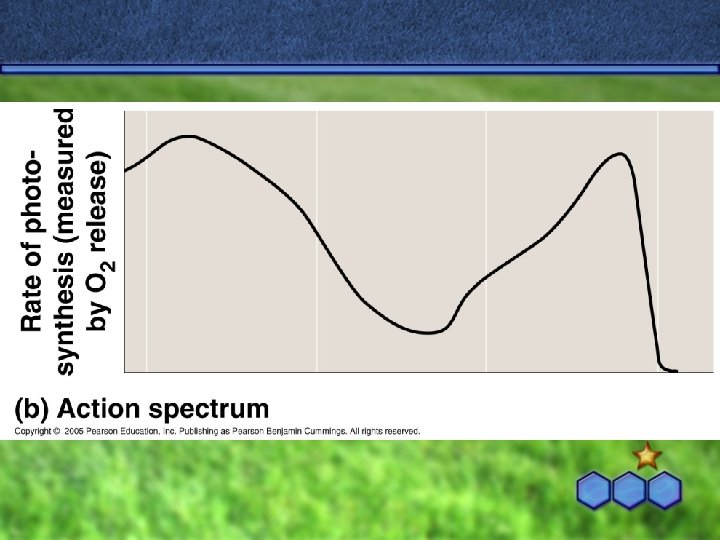
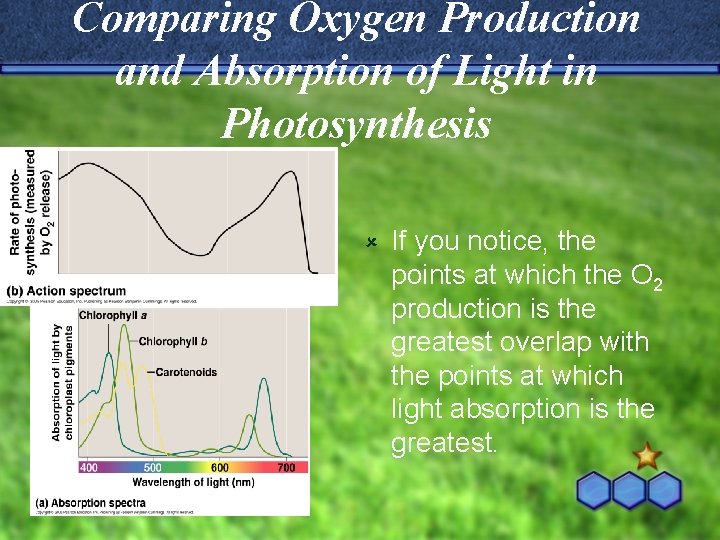
Comparing Oxygen Production and Absorption of Light in Photosynthesis û If you notice, the points at which the O 2 production is the greatest overlap with the points at which light absorption is the greatest.
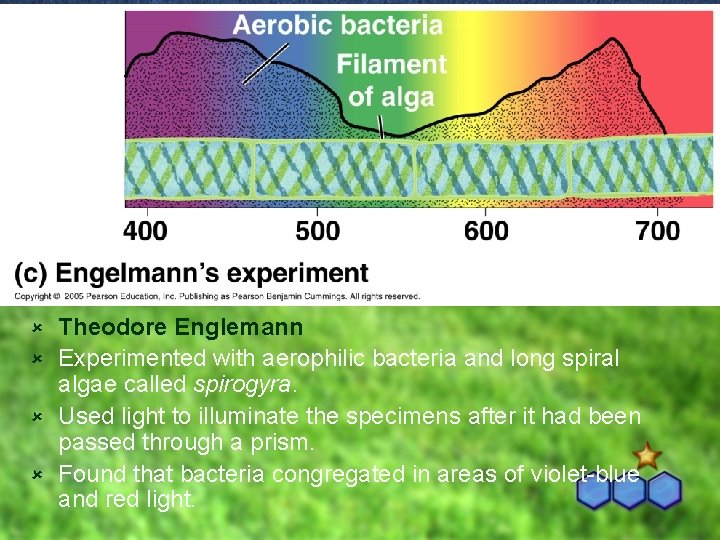
Theodore Englemann û Experimented with aerophilic bacteria and long spiral algae called spirogyra. û Used light to illuminate the specimens after it had been passed through a prism. û Found that bacteria congregated in areas of violet-blue and red light. û
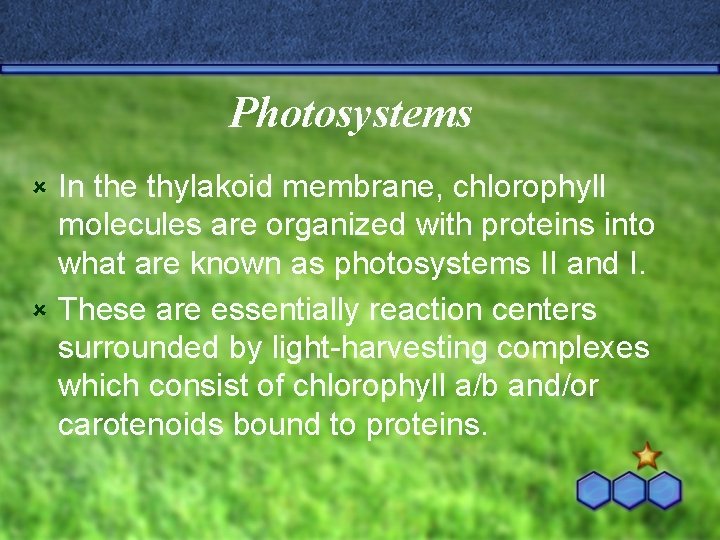
Photosystems In the thylakoid membrane, chlorophyll molecules are organized with proteins into what are known as photosystems II and I. û These are essentially reaction centers surrounded by light-harvesting complexes which consist of chlorophyll a/b and/or carotenoids bound to proteins. û
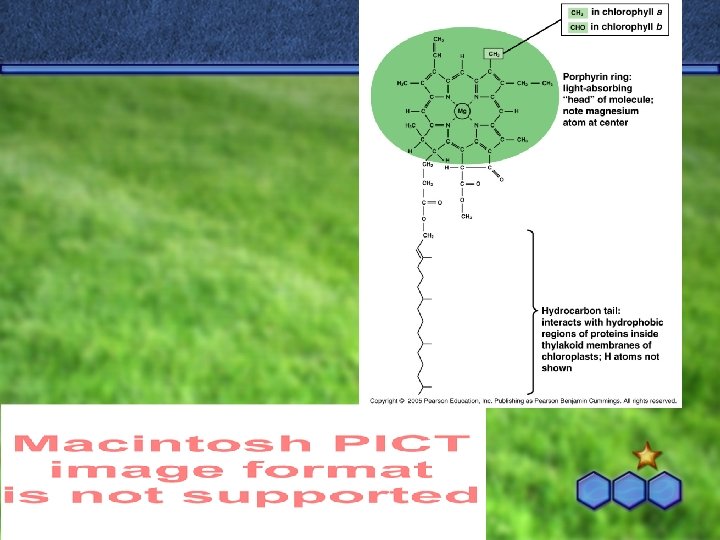
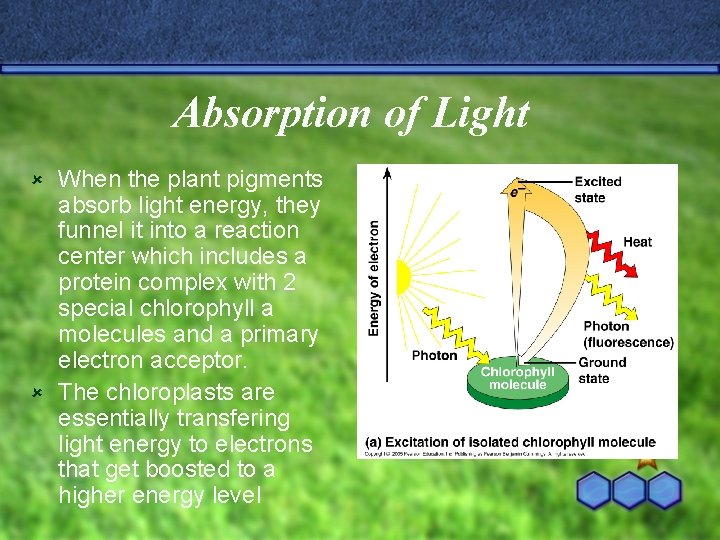
Absorption of Light When the plant pigments absorb light energy, they funnel it into a reaction center which includes a protein complex with 2 special chlorophyll a molecules and a primary electron acceptor. û The chloroplasts are essentially transfering light energy to electrons that get boosted to a higher energy level û
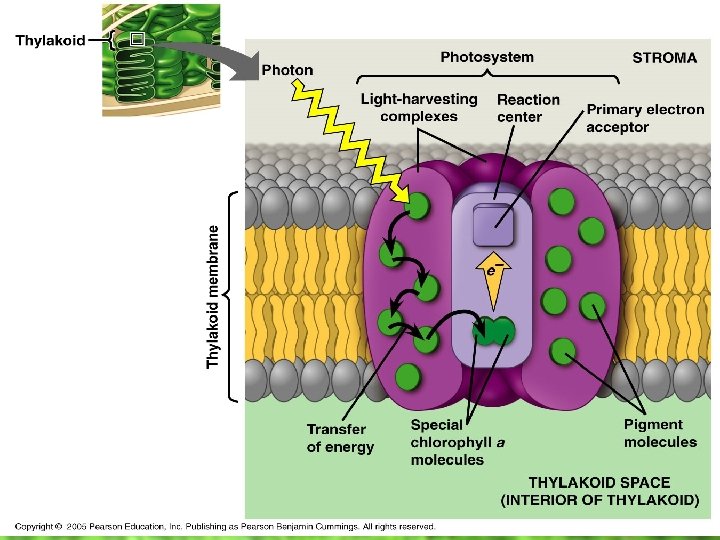
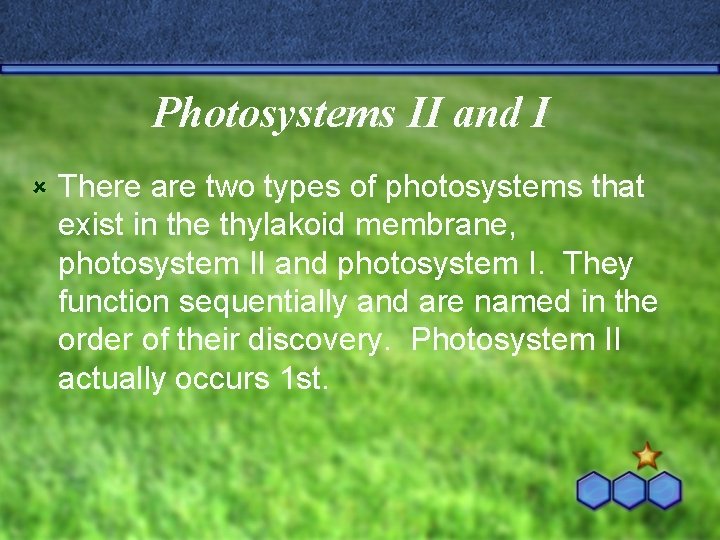
Photosystems II and I û There are two types of photosystems that exist in the thylakoid membrane, photosystem II and photosystem I. They function sequentially and are named in the order of their discovery. Photosystem II actually occurs 1 st.
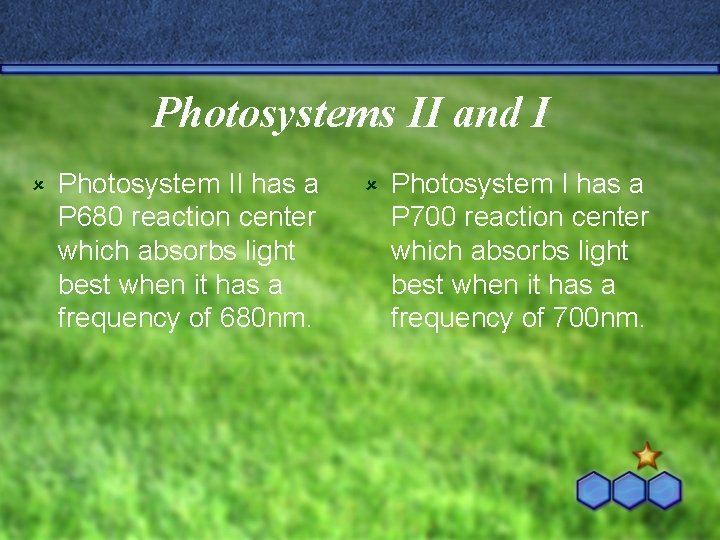
Photosystems II and I û Photosystem II has a P 680 reaction center which absorbs light best when it has a frequency of 680 nm. û Photosystem I has a P 700 reaction center which absorbs light best when it has a frequency of 700 nm.
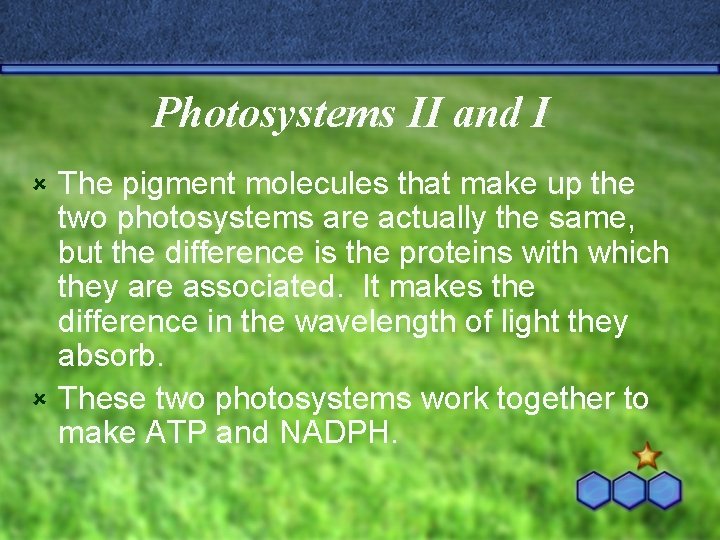
Photosystems II and I The pigment molecules that make up the two photosystems are actually the same, but the difference is the proteins with which they are associated. It makes the difference in the wavelength of light they absorb. û These two photosystems work together to make ATP and NADPH. û
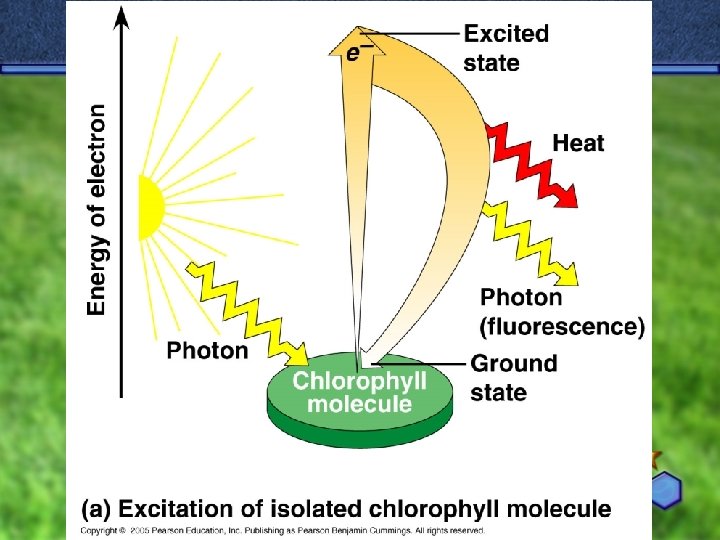
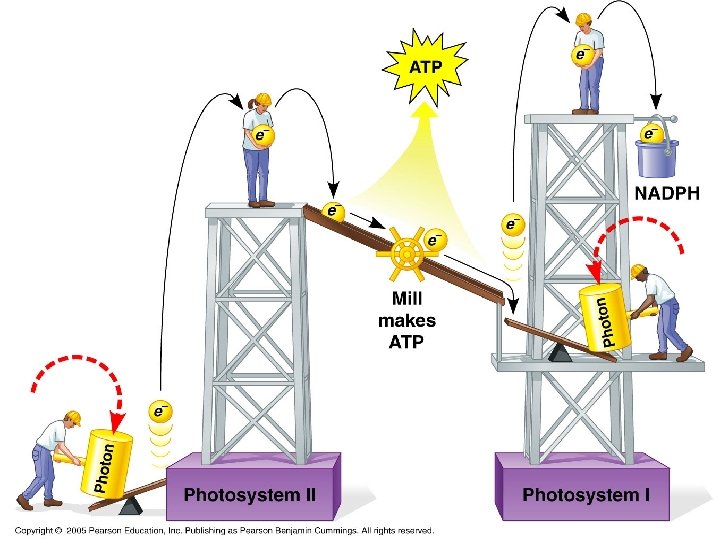
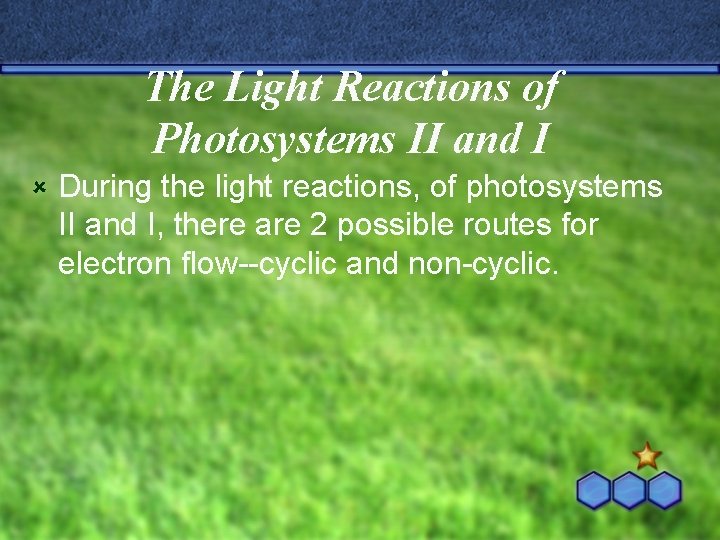
The Light Reactions of Photosystems II and I û During the light reactions, of photosystems II and I, there are 2 possible routes for electron flow--cyclic and non-cyclic.
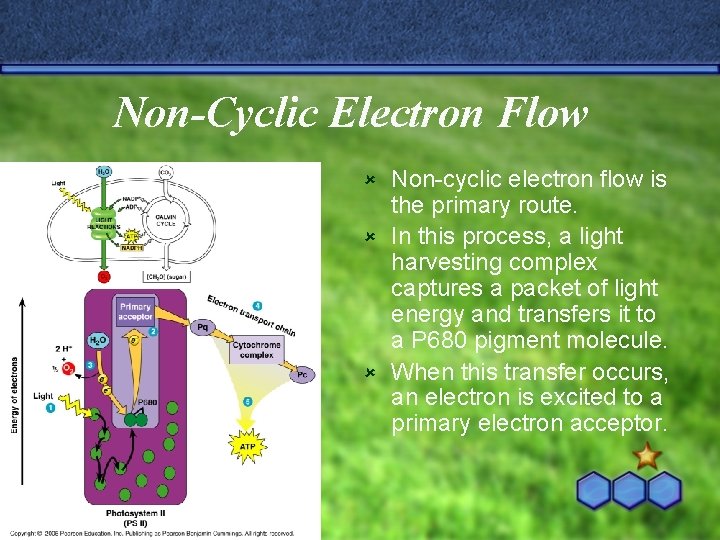
Non-Cyclic Electron Flow Non-cyclic electron flow is the primary route. û In this process, a light harvesting complex captures a packet of light energy and transfers it to a P 680 pigment molecule. û When this transfer occurs, an electron is excited to a primary electron acceptor. û
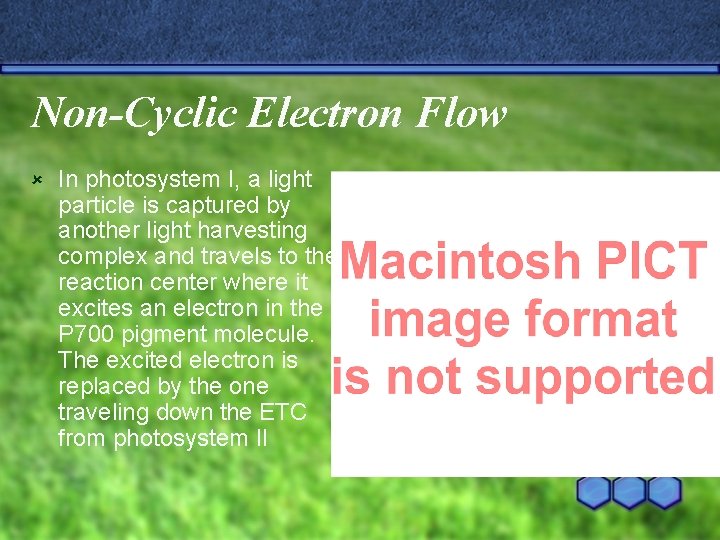
Non-Cyclic Electron Flow û In photosystem I, a light particle is captured by another light harvesting complex and travels to the reaction center where it excites an electron in the P 700 pigment molecule. The excited electron is replaced by the one traveling down the ETC from photosystem II
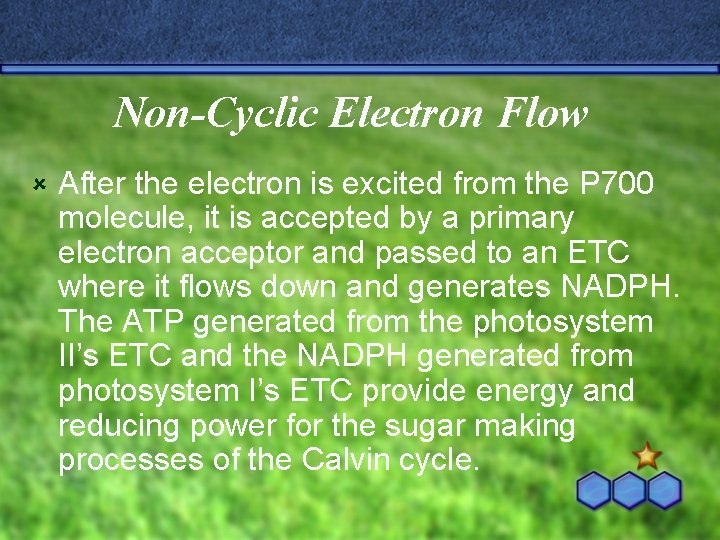
Non-Cyclic Electron Flow û After the electron is excited from the P 700 molecule, it is accepted by a primary electron acceptor and passed to an ETC where it flows down and generates NADPH. The ATP generated from the photosystem II’s ETC and the NADPH generated from photosystem I’s ETC provide energy and reducing power for the sugar making processes of the Calvin cycle.
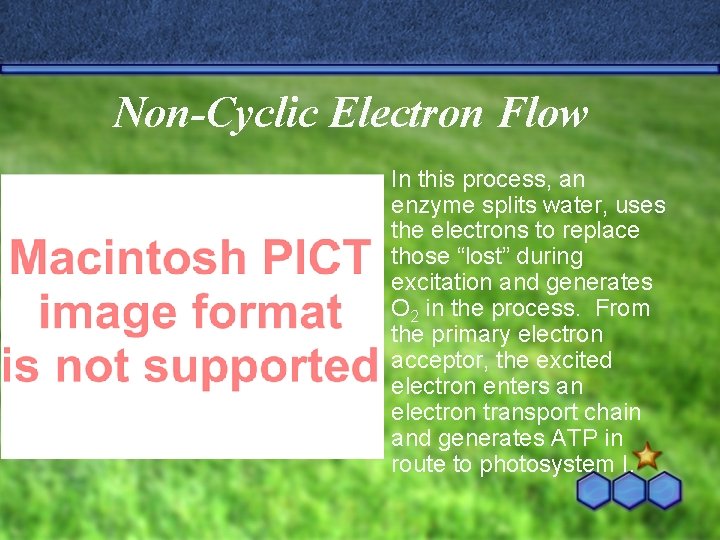
Non-Cyclic Electron Flow û In this process, an enzyme splits water, uses the electrons to replace those “lost” during excitation and generates O 2 in the process. From the primary electron acceptor, the excited electron enters an electron transport chain and generates ATP in route to photosystem I.
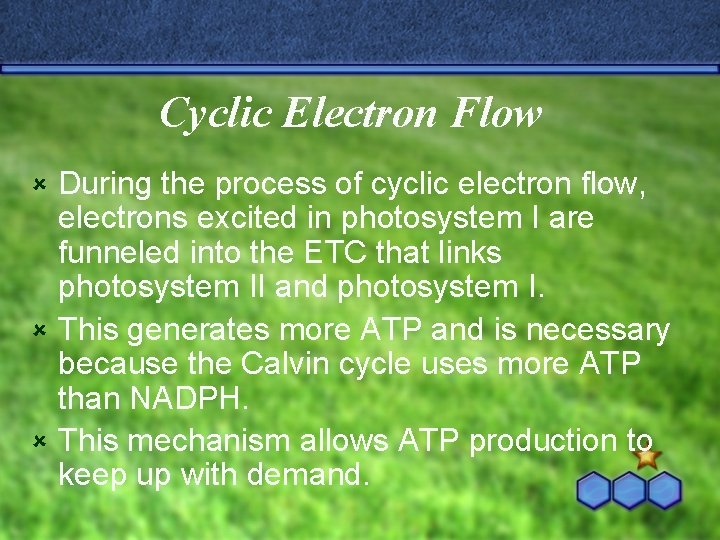
Cyclic Electron Flow During the process of cyclic electron flow, electrons excited in photosystem I are funneled into the ETC that links photosystem II and photosystem I. û This generates more ATP and is necessary because the Calvin cycle uses more ATP than NADPH. û This mechanism allows ATP production to keep up with demand. û
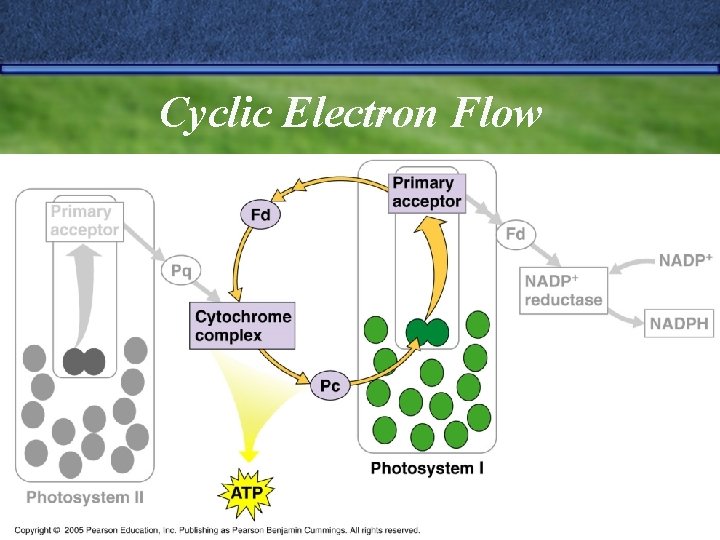
Cyclic Electron Flow
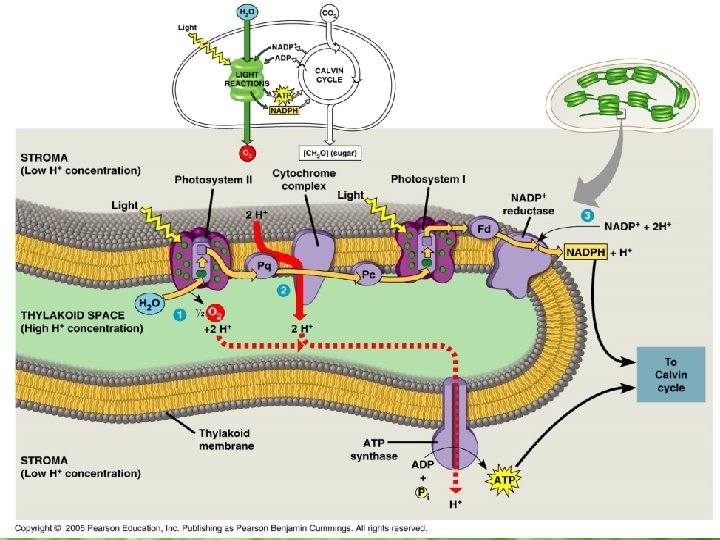
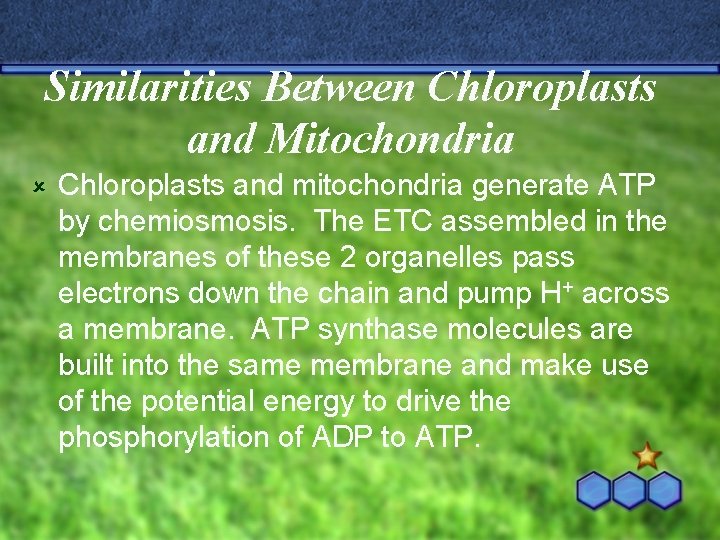
Similarities Between Chloroplasts and Mitochondria û Chloroplasts and mitochondria generate ATP by chemiosmosis. The ETC assembled in the membranes of these 2 organelles pass electrons down the chain and pump H+ across a membrane. ATP synthase molecules are built into the same membrane and make use of the potential energy to drive the phosphorylation of ADP to ATP.
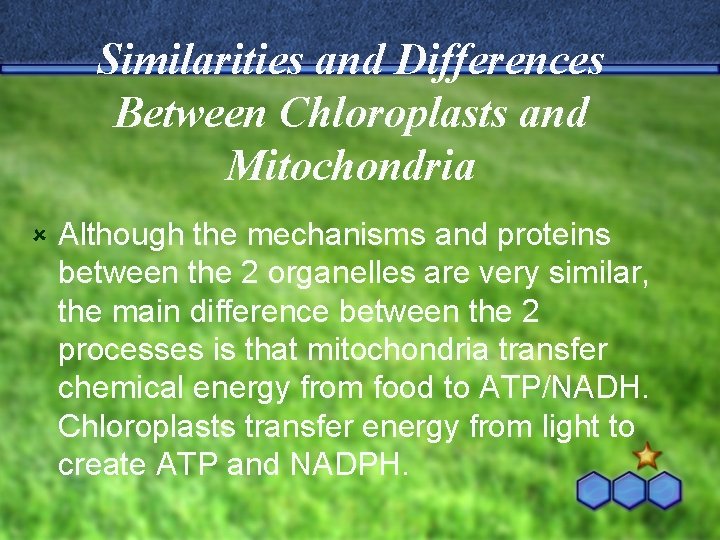
Similarities and Differences Between Chloroplasts and Mitochondria û Although the mechanisms and proteins between the 2 organelles are very similar, the main difference between the 2 processes is that mitochondria transfer chemical energy from food to ATP/NADH. Chloroplasts transfer energy from light to create ATP and NADPH.
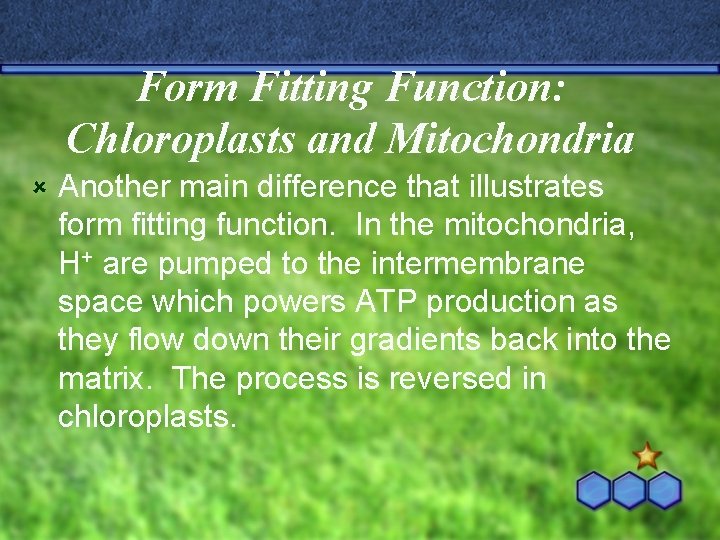
Form Fitting Function: Chloroplasts and Mitochondria û Another main difference that illustrates form fitting function. In the mitochondria, H+ are pumped to the intermembrane space which powers ATP production as they flow down their gradients back into the matrix. The process is reversed in chloroplasts.
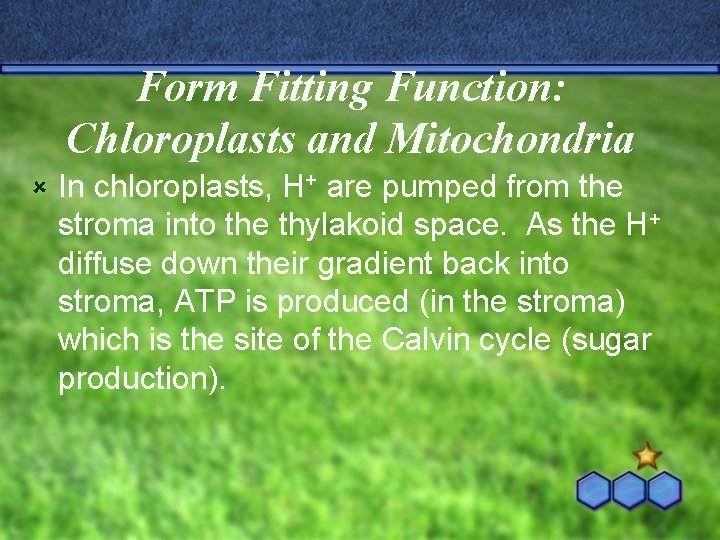
Form Fitting Function: Chloroplasts and Mitochondria û In chloroplasts, H+ are pumped from the stroma into the thylakoid space. As the H+ diffuse down their gradient back into stroma, ATP is produced (in the stroma) which is the site of the Calvin cycle (sugar production).
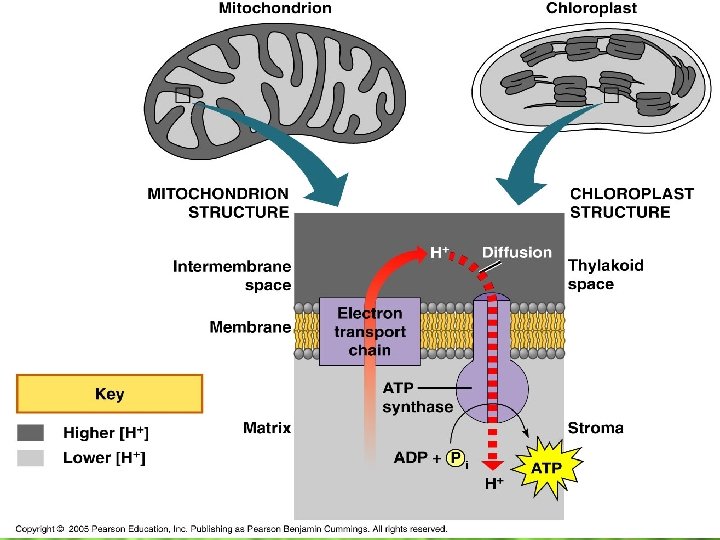
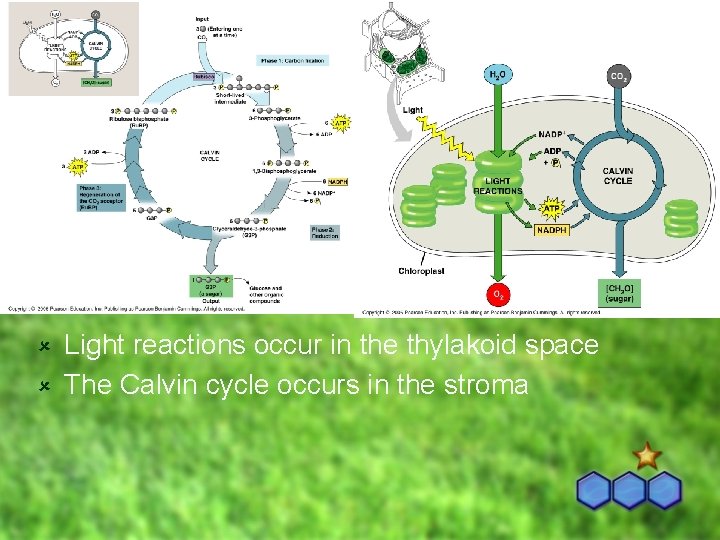
Light reactions occur in the thylakoid space û The Calvin cycle occurs in the stroma û
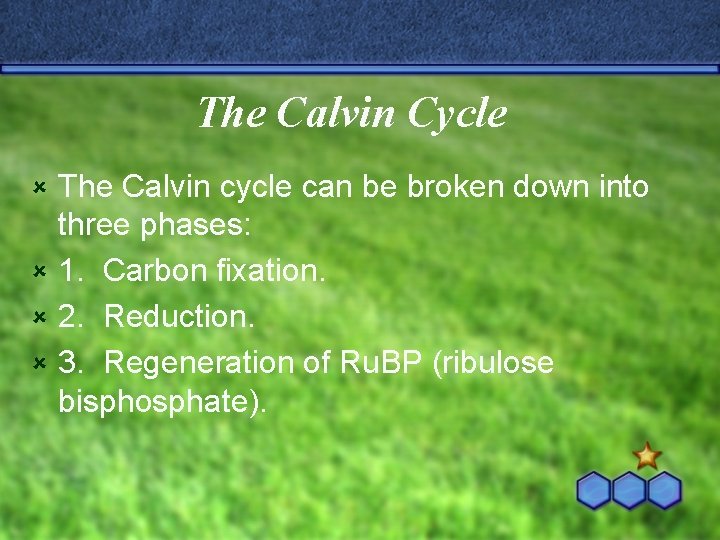
The Calvin Cycle The Calvin cycle can be broken down into three phases: û 1. Carbon fixation. û 2. Reduction. û 3. Regeneration of Ru. BP (ribulose bisphosphate). û
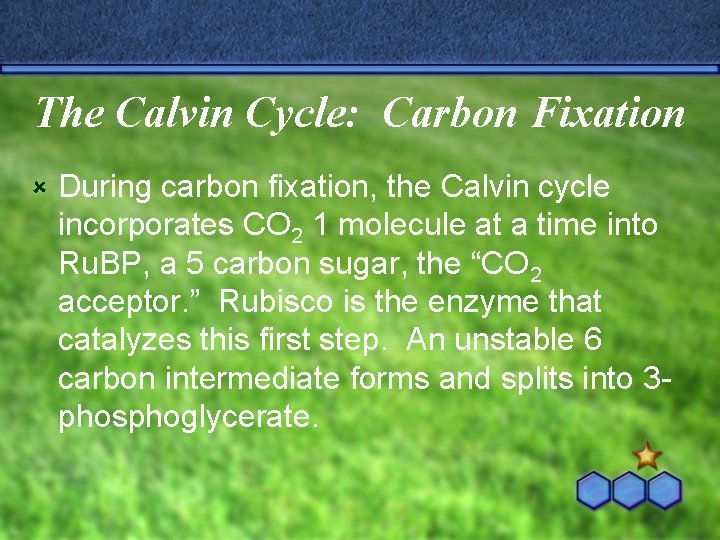
The Calvin Cycle: Carbon Fixation û During carbon fixation, the Calvin cycle incorporates CO 2 1 molecule at a time into Ru. BP, a 5 carbon sugar, the “CO 2 acceptor. ” Rubisco is the enzyme that catalyzes this first step. An unstable 6 carbon intermediate forms and splits into 3 phosphoglycerate.
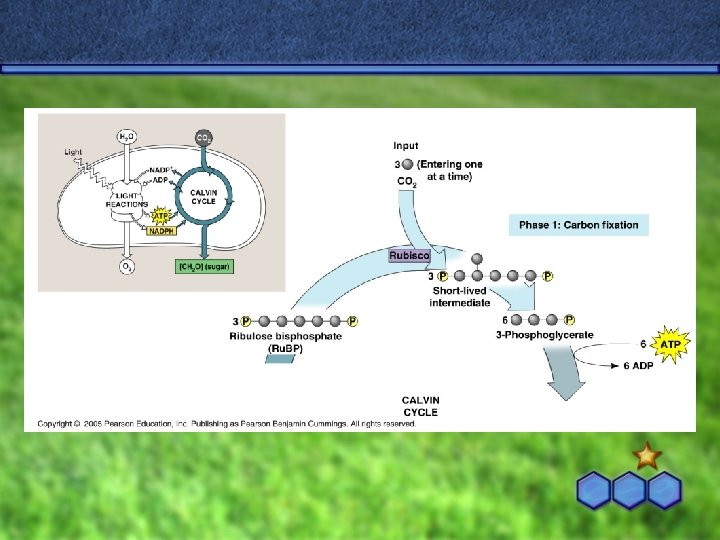
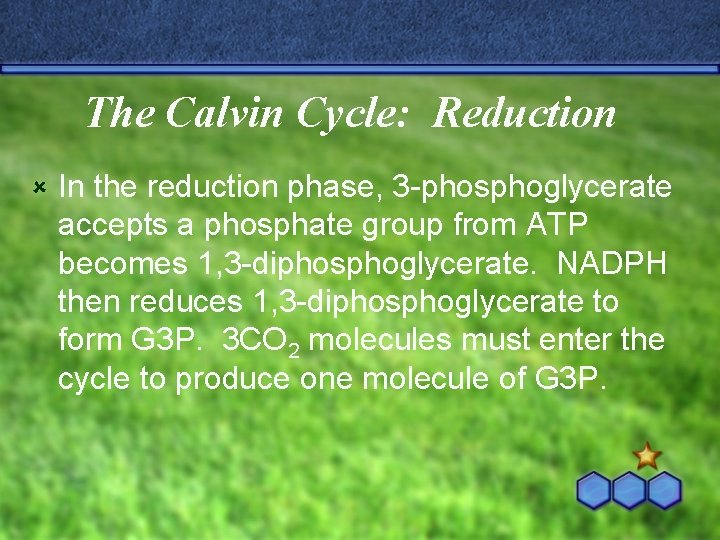
The Calvin Cycle: Reduction û In the reduction phase, 3 -phosphoglycerate accepts a phosphate group from ATP becomes 1, 3 -diphosphoglycerate. NADPH then reduces 1, 3 -diphosphoglycerate to form G 3 P. 3 CO 2 molecules must enter the cycle to produce one molecule of G 3 P.
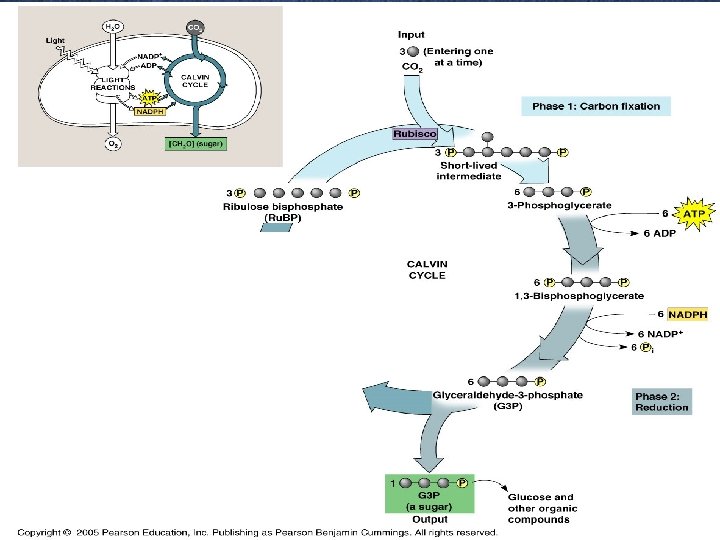
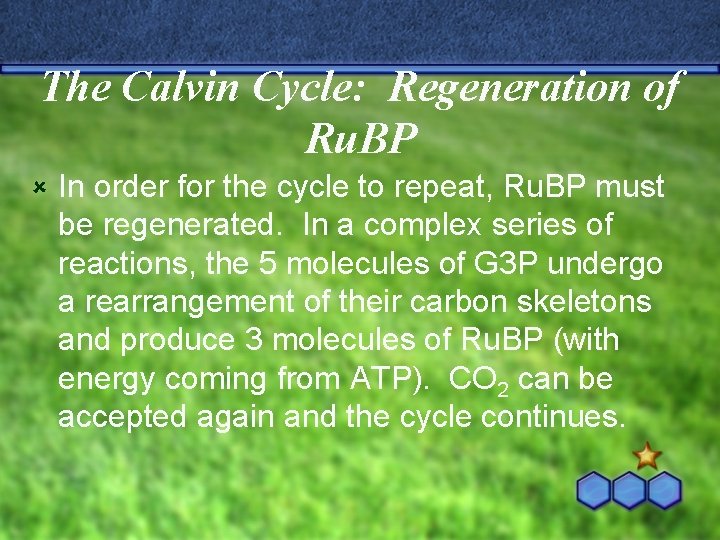
The Calvin Cycle: Regeneration of Ru. BP û In order for the cycle to repeat, Ru. BP must be regenerated. In a complex series of reactions, the 5 molecules of G 3 P undergo a rearrangement of their carbon skeletons and produce 3 molecules of Ru. BP (with energy coming from ATP). CO 2 can be accepted again and the cycle continues.
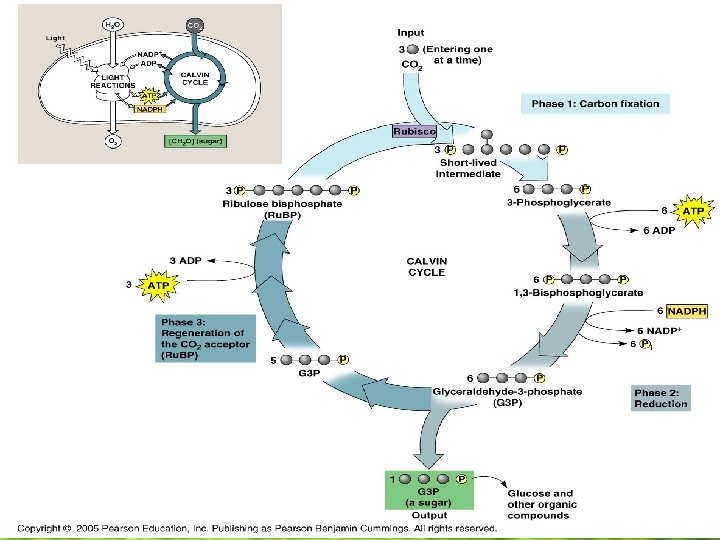
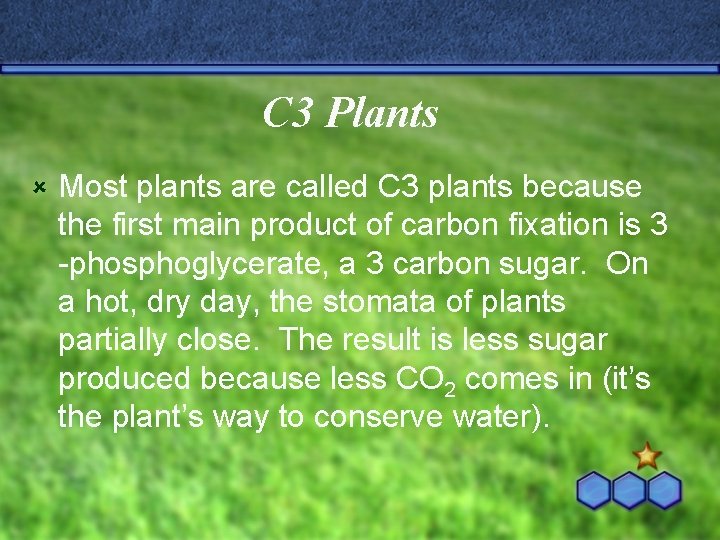
C 3 Plants û Most plants are called C 3 plants because the first main product of carbon fixation is 3 -phosphoglycerate, a 3 carbon sugar. On a hot, dry day, the stomata of plants partially close. The result is less sugar produced because less CO 2 comes in (it’s the plant’s way to conserve water).
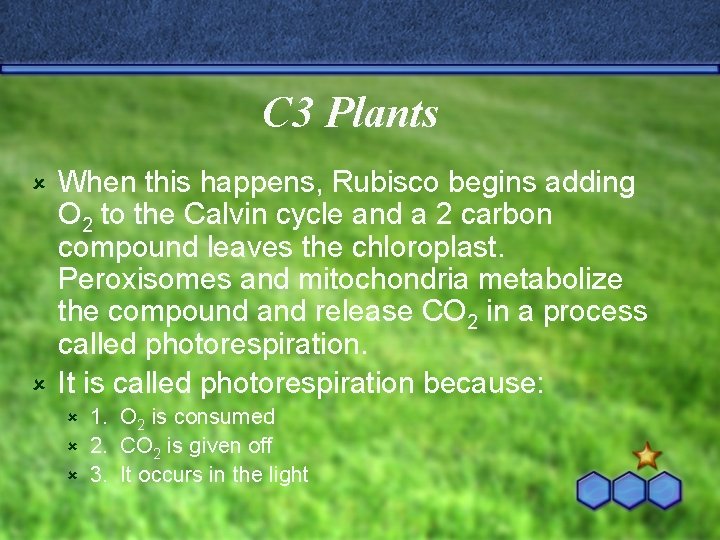
C 3 Plants When this happens, Rubisco begins adding O 2 to the Calvin cycle and a 2 carbon compound leaves the chloroplast. Peroxisomes and mitochondria metabolize the compound and release CO 2 in a process called photorespiration. û It is called photorespiration because: û 1. O 2 is consumed û 2. CO 2 is given off û 3. It occurs in the light û
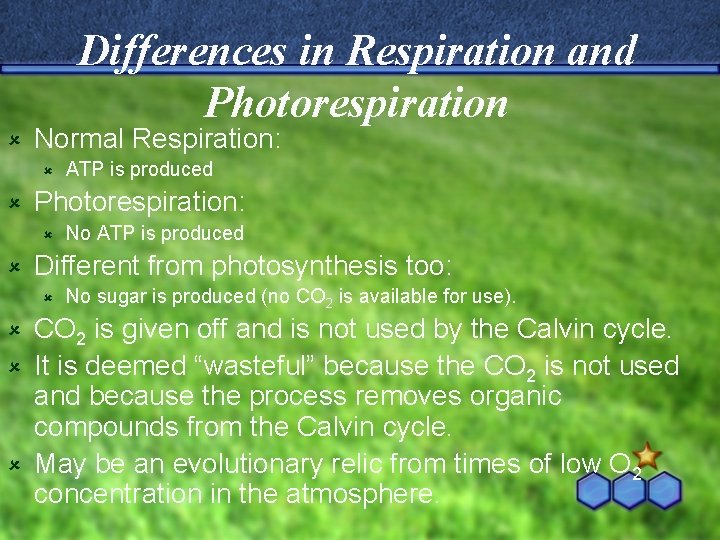
Differences in Respiration and Photorespiration û Normal Respiration: û û Photorespiration: û û ATP is produced No ATP is produced Different from photosynthesis too: û No sugar is produced (no CO 2 is available for use). CO 2 is given off and is not used by the Calvin cycle. û It is deemed “wasteful” because the CO 2 is not used and because the process removes organic compounds from the Calvin cycle. û May be an evolutionary relic from times of low O 2 concentration in the atmosphere. û
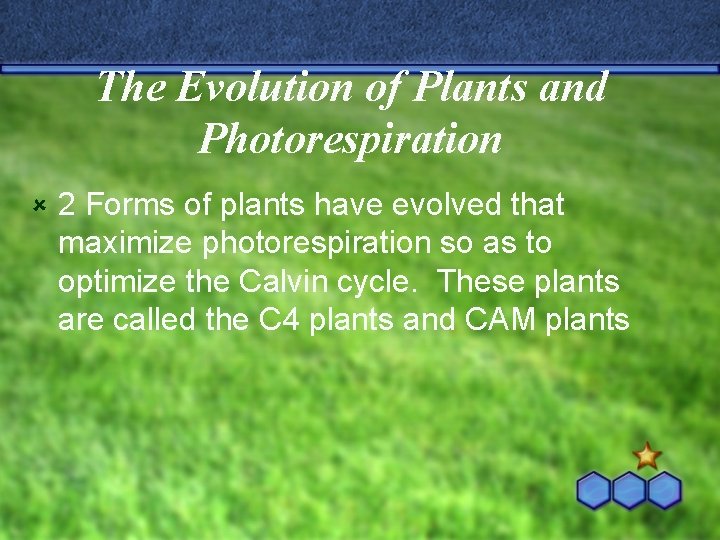
The Evolution of Plants and Photorespiration û 2 Forms of plants have evolved that maximize photorespiration so as to optimize the Calvin cycle. These plants are called the C 4 plants and CAM plants
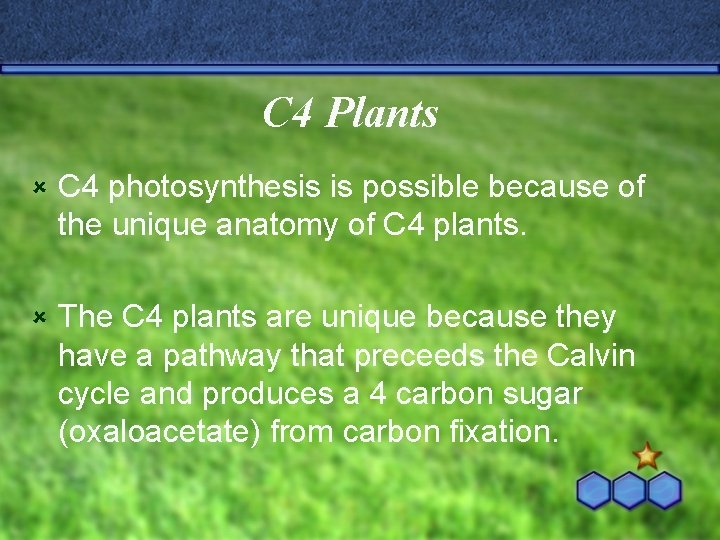
C 4 Plants û C 4 photosynthesis is possible because of the unique anatomy of C 4 plants. û The C 4 plants are unique because they have a pathway that preceeds the Calvin cycle and produces a 4 carbon sugar (oxaloacetate) from carbon fixation.
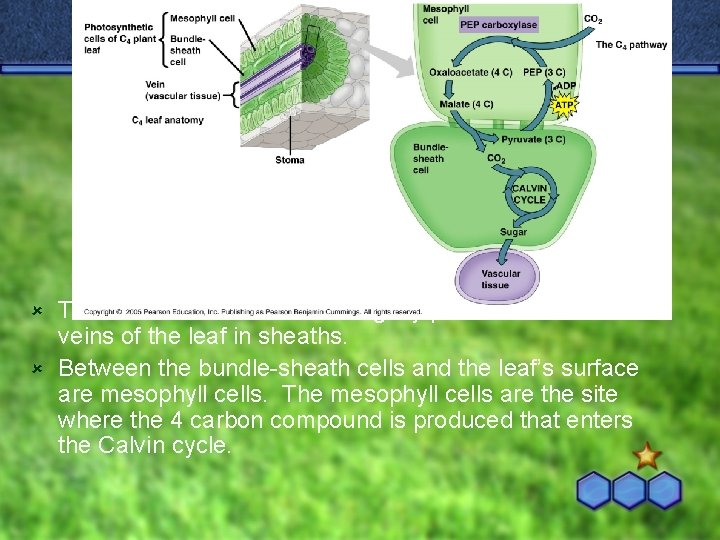
The bundle-sheath cells are tightly packed around the veins of the leaf in sheaths. û Between the bundle-sheath cells and the leaf’s surface are mesophyll cells. The mesophyll cells are the site where the 4 carbon compound is produced that enters the Calvin cycle. û
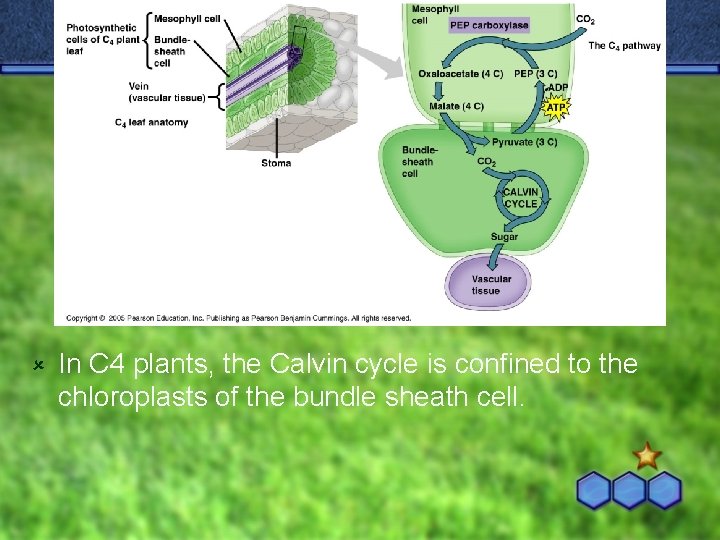
û In C 4 plants, the Calvin cycle is confined to the chloroplasts of the bundle sheath cell.
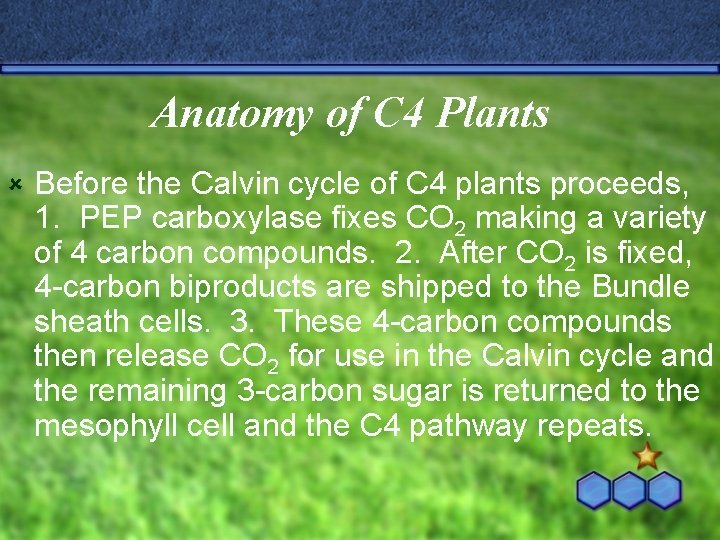
Anatomy of C 4 Plants û Before the Calvin cycle of C 4 plants proceeds, 1. PEP carboxylase fixes CO 2 making a variety of 4 carbon compounds. 2. After CO 2 is fixed, 4 -carbon biproducts are shipped to the Bundle sheath cells. 3. These 4 -carbon compounds then release CO 2 for use in the Calvin cycle and the remaining 3 -carbon sugar is returned to the mesophyll cell and the C 4 pathway repeats.
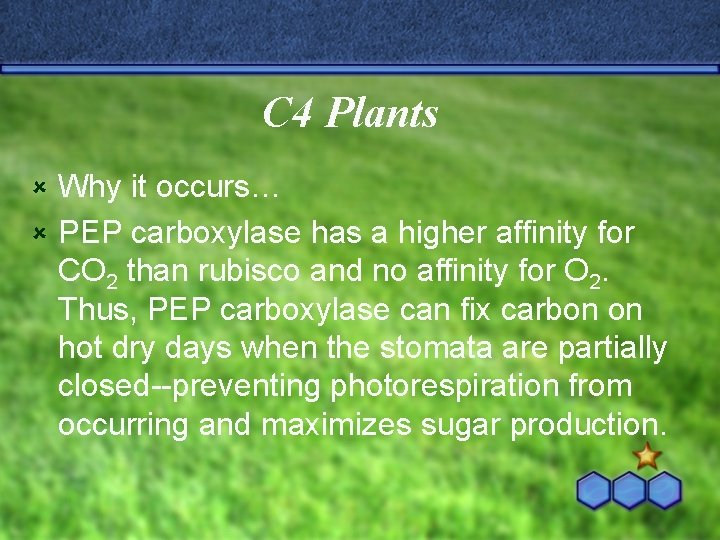
C 4 Plants Why it occurs… û PEP carboxylase has a higher affinity for CO 2 than rubisco and no affinity for O 2. Thus, PEP carboxylase can fix carbon on hot dry days when the stomata are partially closed--preventing photorespiration from occurring and maximizes sugar production. û
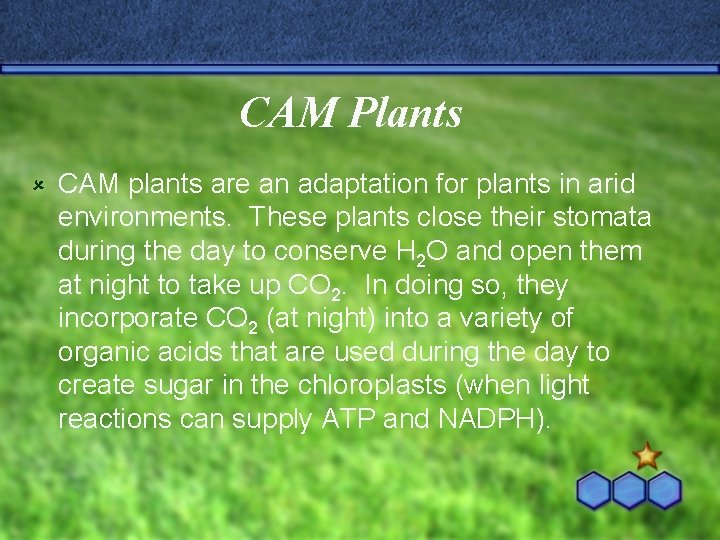
CAM Plants û CAM plants are an adaptation for plants in arid environments. These plants close their stomata during the day to conserve H 2 O and open them at night to take up CO 2. In doing so, they incorporate CO 2 (at night) into a variety of organic acids that are used during the day to create sugar in the chloroplasts (when light reactions can supply ATP and NADPH).
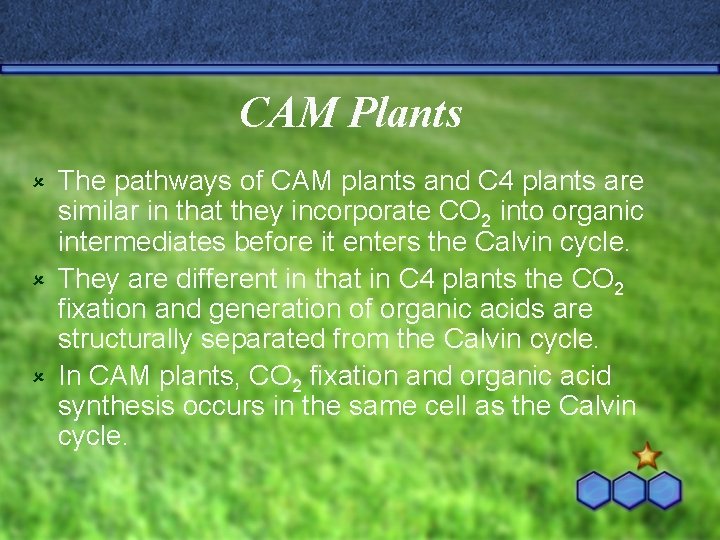
CAM Plants The pathways of CAM plants and C 4 plants are similar in that they incorporate CO 2 into organic intermediates before it enters the Calvin cycle. û They are different in that in C 4 plants the CO 2 fixation and generation of organic acids are structurally separated from the Calvin cycle. û In CAM plants, CO 2 fixation and organic acid synthesis occurs in the same cell as the Calvin cycle. û
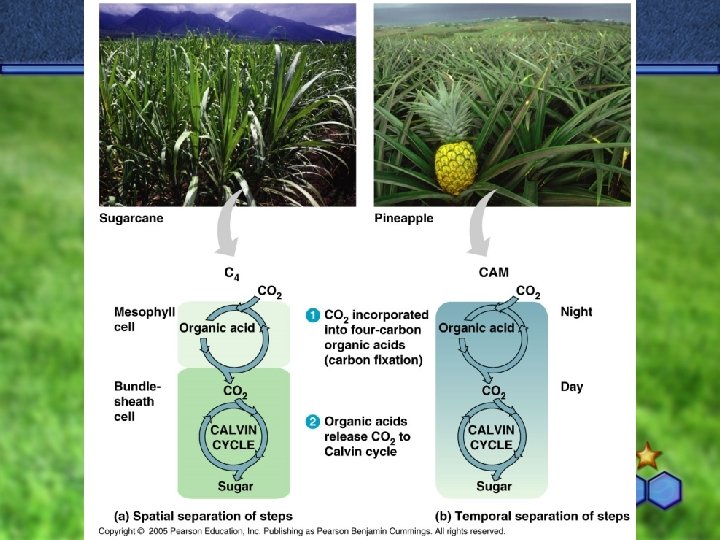
Autotrophs and heterotrophs
Explain how adp and atp are each like a battery
Are cnidarians autotrophs or heterotrophs
Do owls eat
Are fungi autotrophs or heterotrophs
How do sac fungi reproduce
Is eubacteria terrestrial or aquatic
Mikael ferm
Are archaebacteria heterotrophs
Unicellular heterotrophs
Fungi are also called multicellular decomposers
Endangered animals of north america
Unit 1 diversity in the living world
Protozoa are polyphyletic
Saprophytic bacteria
Eukaryotic heterotrophs
Heterotrophs
Rhizopus diagram
All animals are multicellular heterotrophic eukaryotes
Ingestive heterotrophs
Ultimate source of energy in food chain
Heterotrophs
Are fungi single celled or multicellular
Kingdom
Example of heterotrophs
What is the ultimate source of energy in a food chain
Ingestive heterotrophs
Limiting factor examples
Light reactions photosynthesis
Phylum name
Are archaebacteria autotrophs
Autotrophs
Which organism is an autotroph
Chapter 6 cell energy photosynthesis and respiration
Section 8-2 photosynthesis an overview answer key
Cellular energy section 2 photosynthesis
Chapter 9 lesson 2 photosynthesis an overview
Chapter 8 photosynthesis vocabulary review
What are the products of photosynthesis?
The process of photosynthesis
Mcgraw hill photosynthesis
The process of photosynthesis and cellular respiration
Cellular respiration and photosynthesis equation
Photosynthesis and food chain
Formula for celluar respiration
Venn diagram photosynthesis and respiration
Venn diagram photosynthesis and respiration
What are the reactants and products of photosynthesis?
Formula for photosynthesis and cellular respiration
Photosynthesis and cellular respiration diagram
Cellular respiration foldable ap biology
Chemosynthesis vs photosynthesis venn diagram
Plants called sundews have rounded green leaves
Edpuzzle cellular respiration
Describe photosynthesis
Interrelationship between photosynthesis and respiration
Photosynthesis and cellular respiration
Snails and elodea virtual lab
Chlorophyll structure
Photosynthesis in symbols
Photosynthesis and cellular respiration jeopardy
Write the overall equation for photosynthesis
Where does photosynthesis take place
The purpose of photosynthesis
Word equation for photosynthesis
Inputs of light reactions in photosynthesis
The process of photosynthesis step by step
Starchy plants
Euglena and volvox are
Green plants make their own food by photosynthesis
What happens during photosynthesis
Where does the photosynthesis occur
Chemiosmosis in photosynthesis
Photosynthesis poem
What is the word equation of photosynthesis
Photosynthesis song
Chemical equation for cellular respiration
Photosynthesis equation