Weekly Presentations AAE 450 Project Destiny Section 1
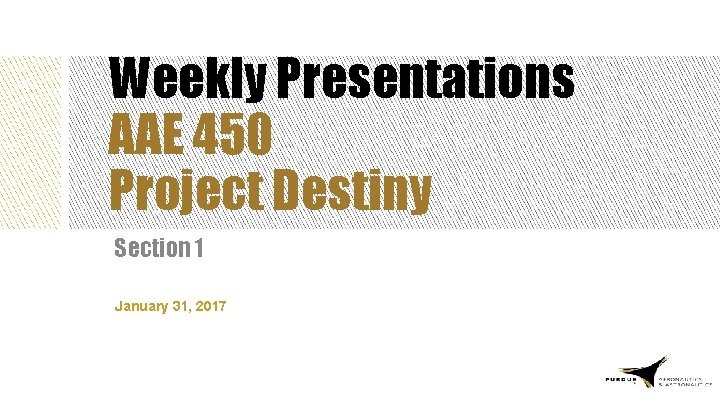
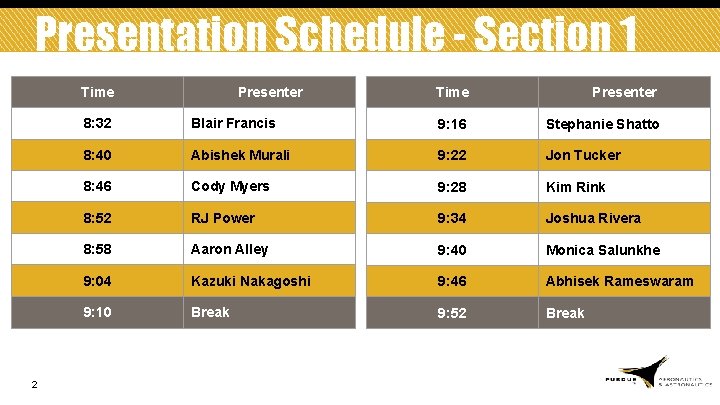
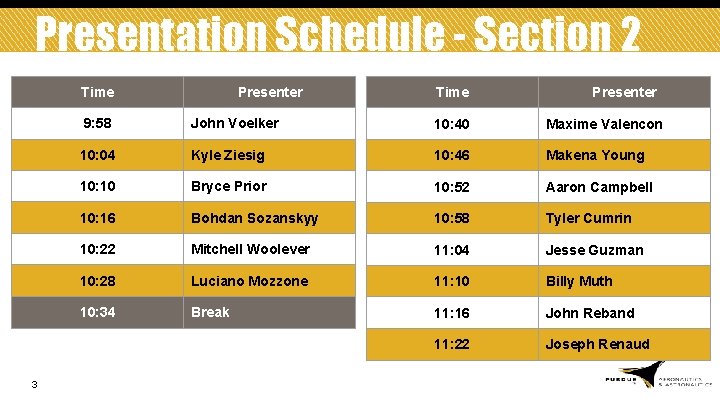
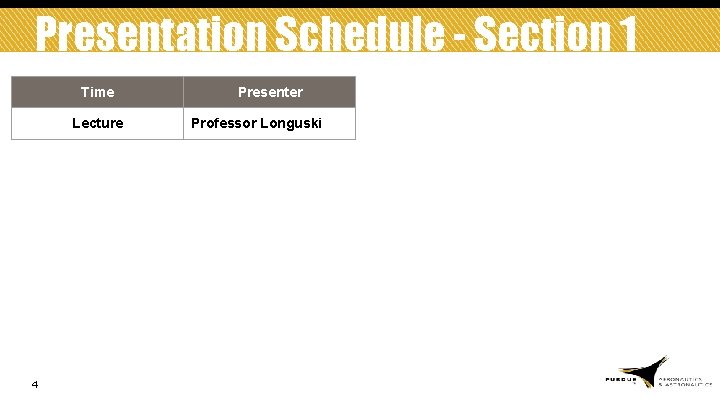
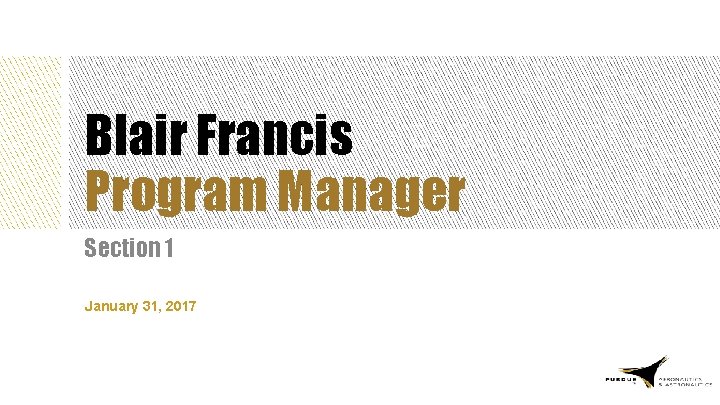
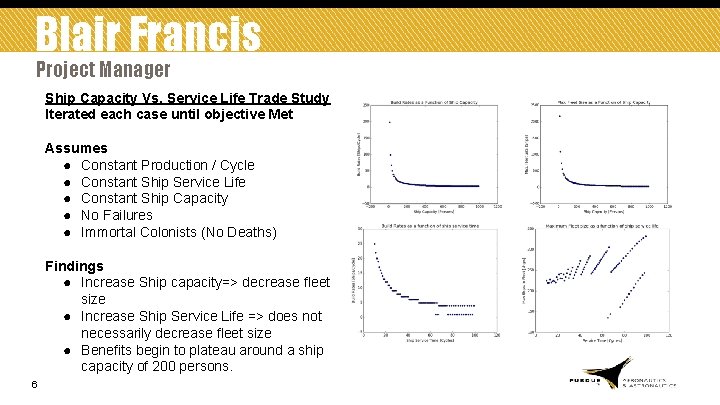
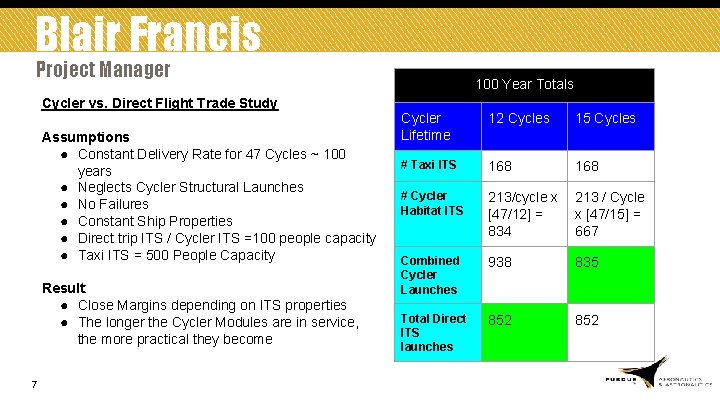
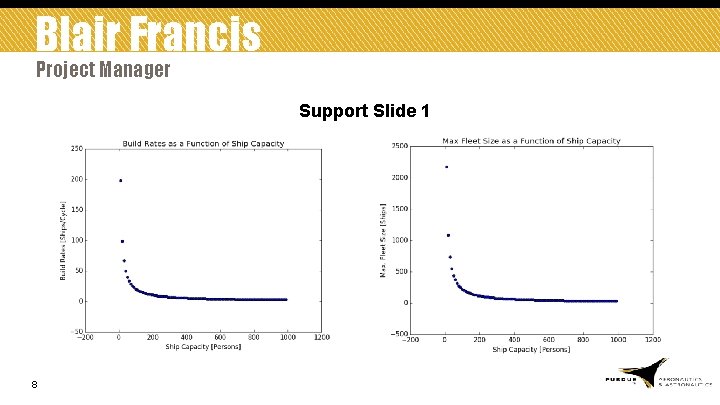
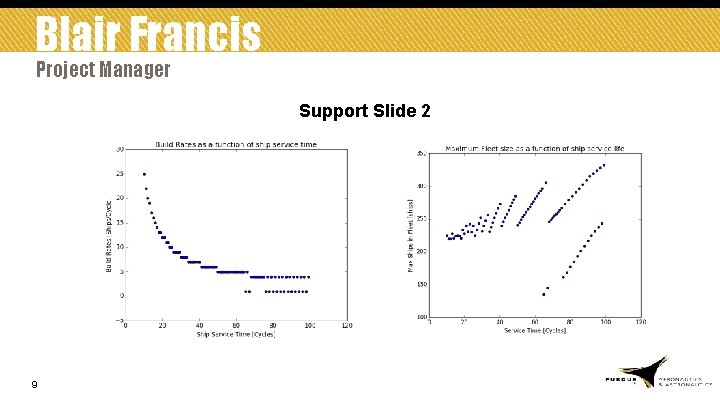
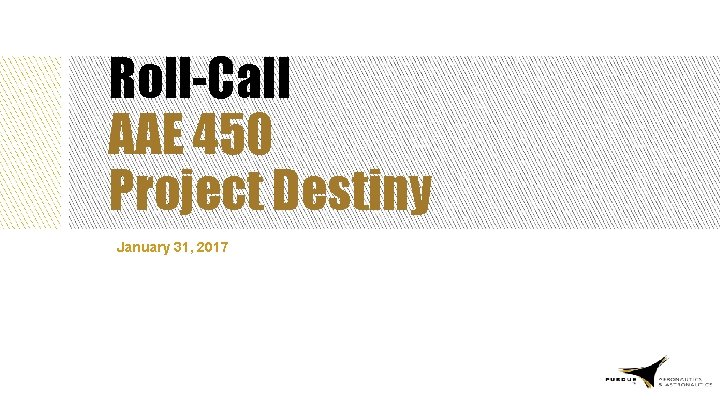
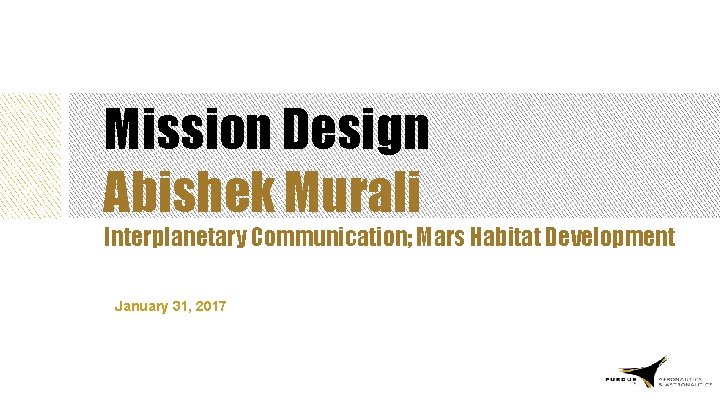
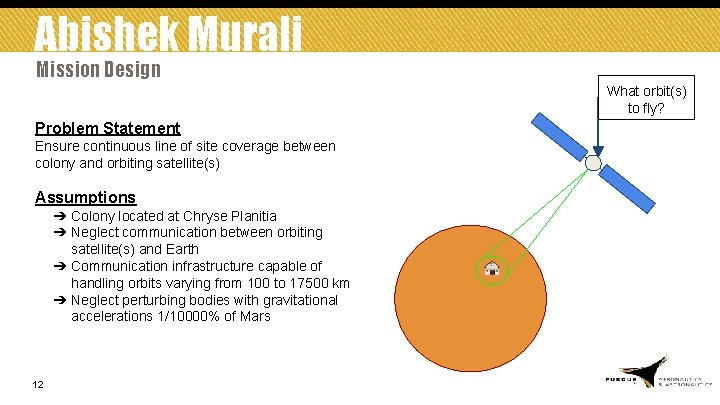
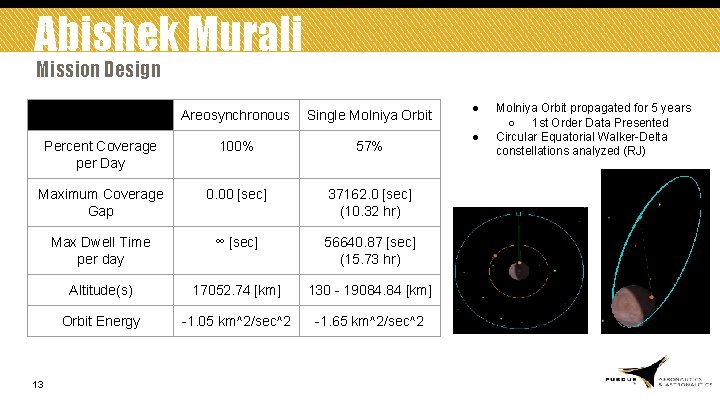
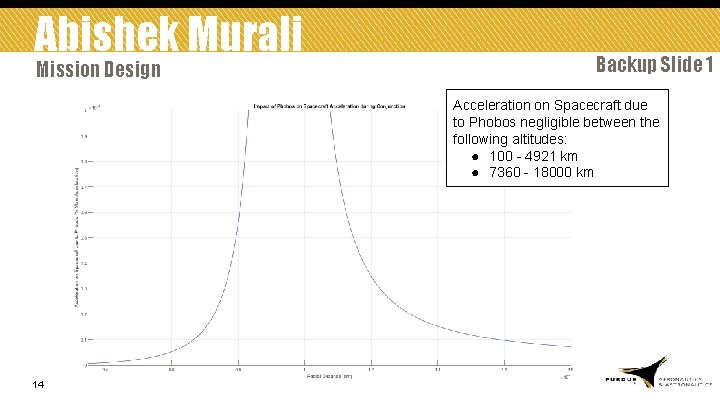
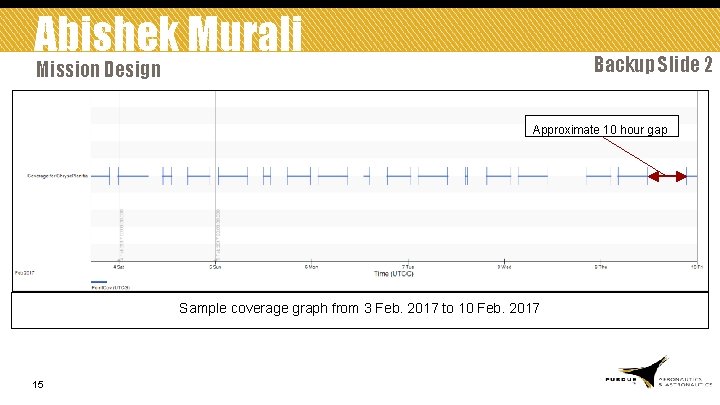
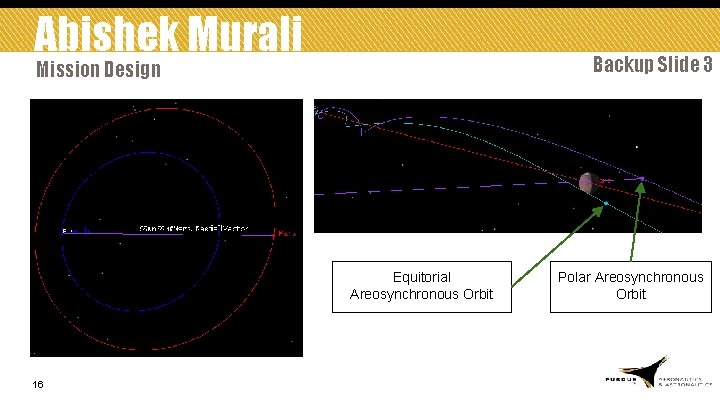
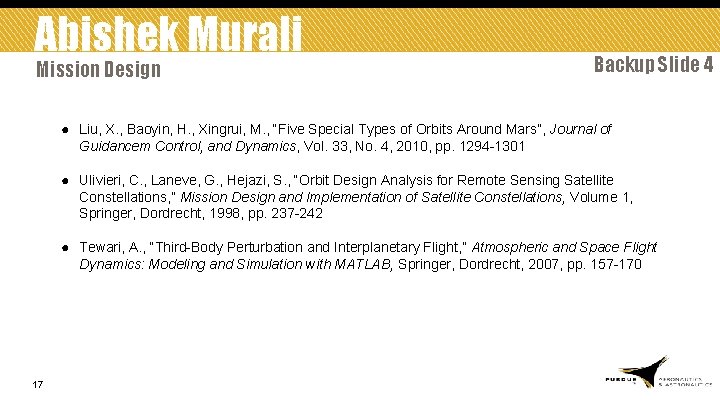
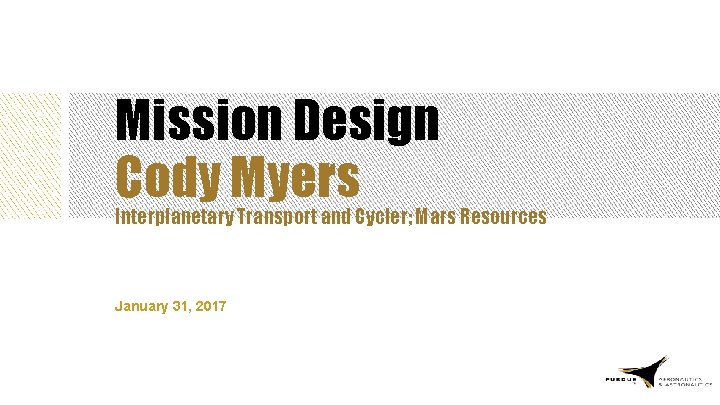
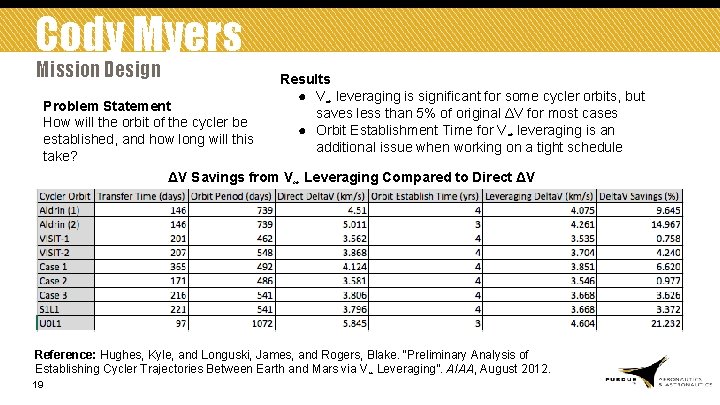
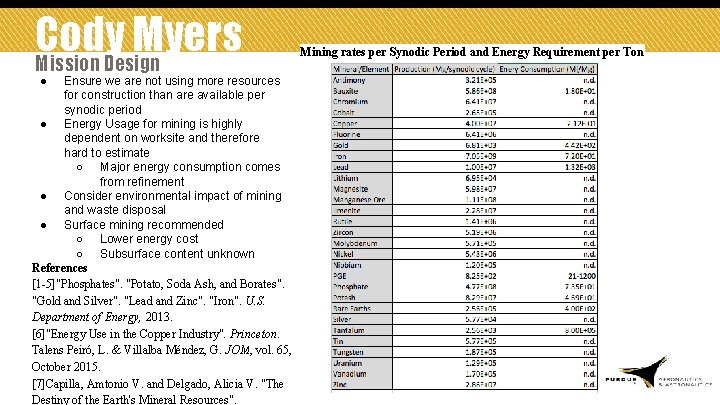
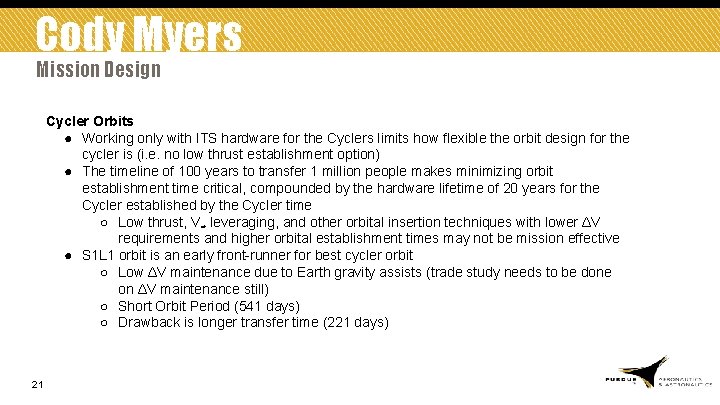
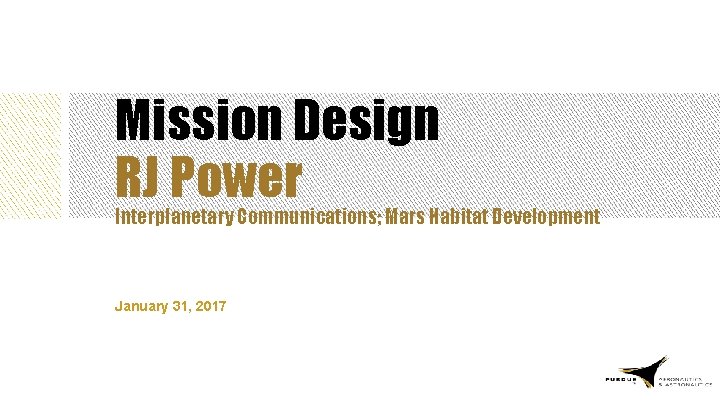
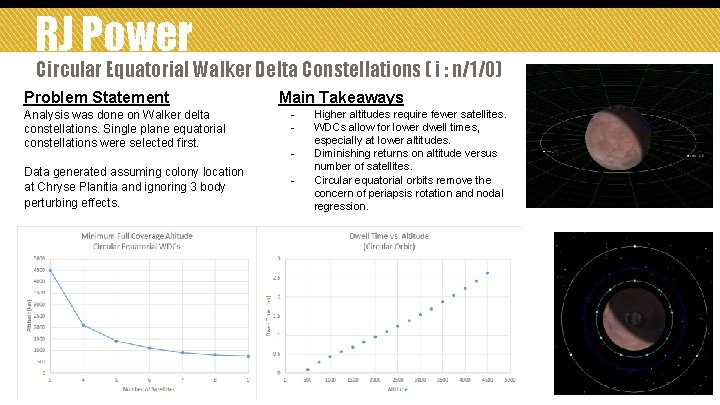
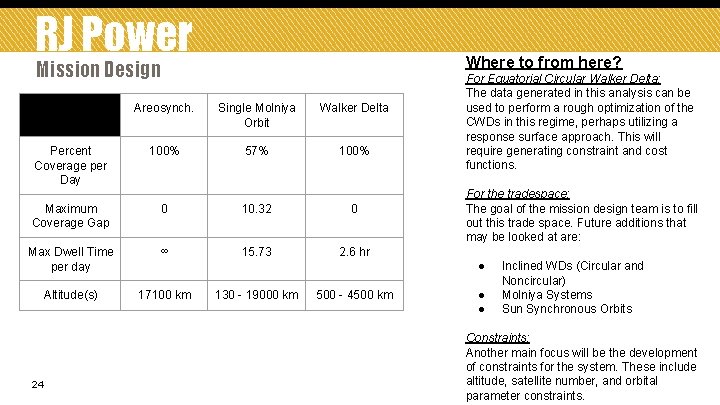
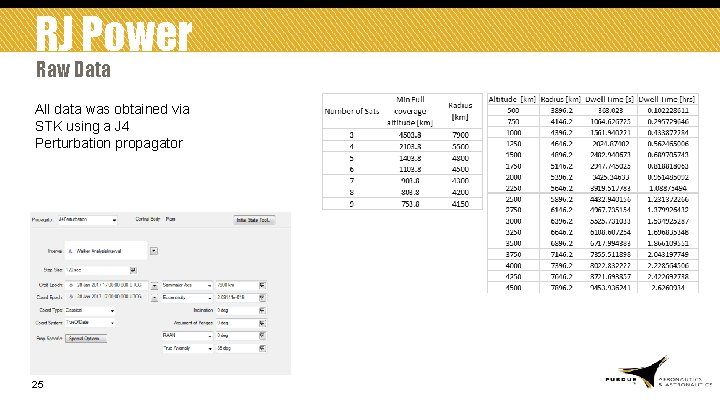
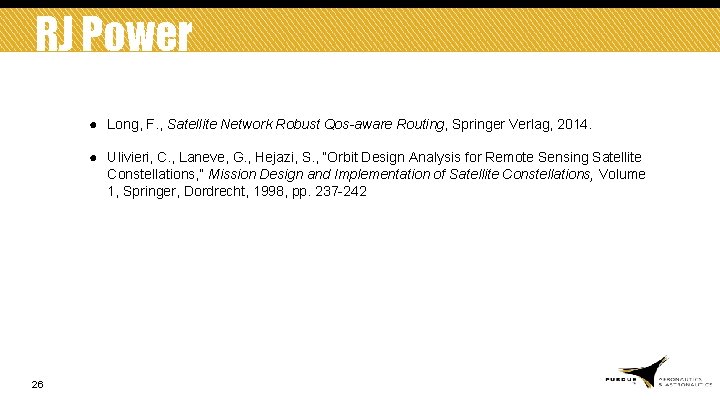
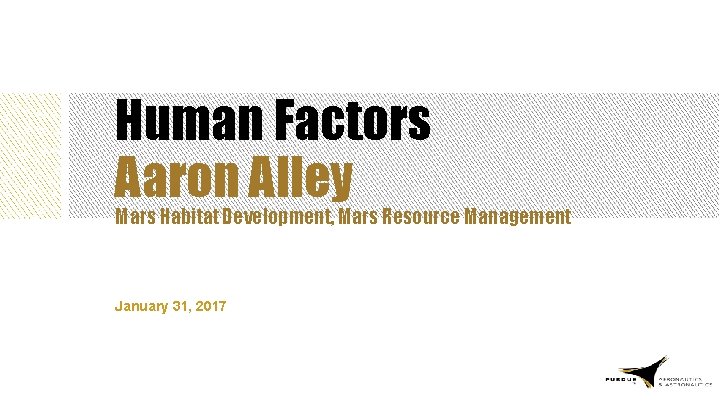
![Aaron Alley Human Factors ● Dorms ○ 9. 6 m^2 [1 -5] ● Purdue Aaron Alley Human Factors ● Dorms ○ 9. 6 m^2 [1 -5] ● Purdue](https://slidetodoc.com/presentation_image_h/184fd3da0ebff5691a469b53cfd00971/image-28.jpg)
![Aaron Alley Human Factors ● Hostel [7] ○ 6. 5 m^2 ● NYC single Aaron Alley Human Factors ● Hostel [7] ○ 6. 5 m^2 ● NYC single](https://slidetodoc.com/presentation_image_h/184fd3da0ebff5691a469b53cfd00971/image-29.jpg)
![Aaron Alley Human Factors [1] "Room Layouts, " Concordia University [online]. http: //www. cune. Aaron Alley Human Factors [1] "Room Layouts, " Concordia University [online]. http: //www. cune.](https://slidetodoc.com/presentation_image_h/184fd3da0ebff5691a469b53cfd00971/image-30.jpg)
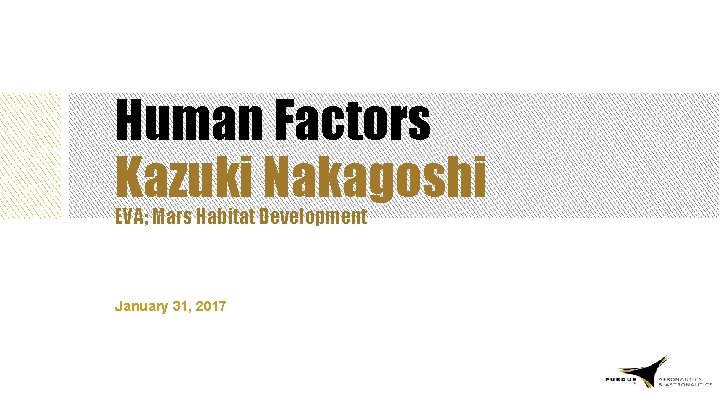
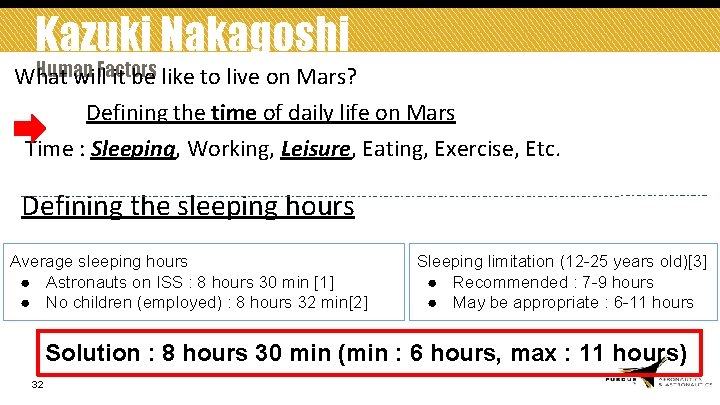
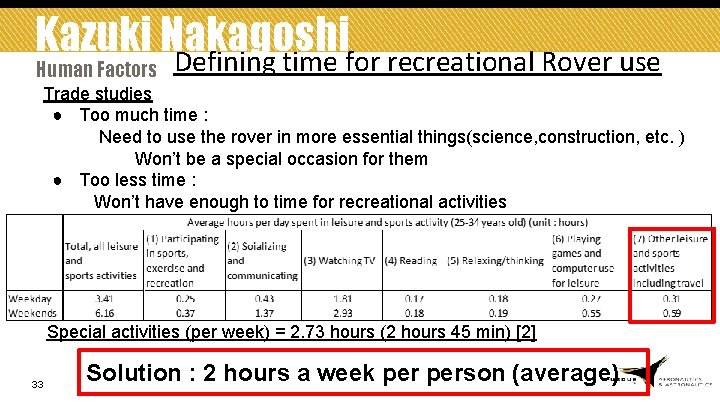
![Kazuki Nakagoshi Human Factors Comparison of daily life between astronaut and nonhousehold employer [1], Kazuki Nakagoshi Human Factors Comparison of daily life between astronaut and nonhousehold employer [1],](https://slidetodoc.com/presentation_image_h/184fd3da0ebff5691a469b53cfd00971/image-34.jpg)
![Kazuki Nakagoshi Human Factors Reference [1]NASA, “International Space Station Timelines” Jan. 16, 2014 [online Kazuki Nakagoshi Human Factors Reference [1]NASA, “International Space Station Timelines” Jan. 16, 2014 [online](https://slidetodoc.com/presentation_image_h/184fd3da0ebff5691a469b53cfd00971/image-35.jpg)
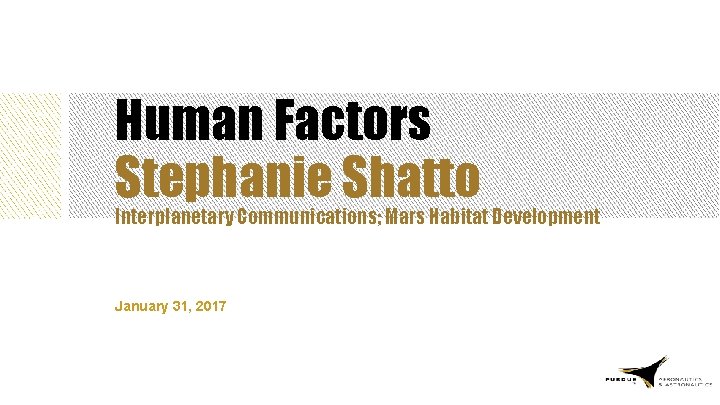
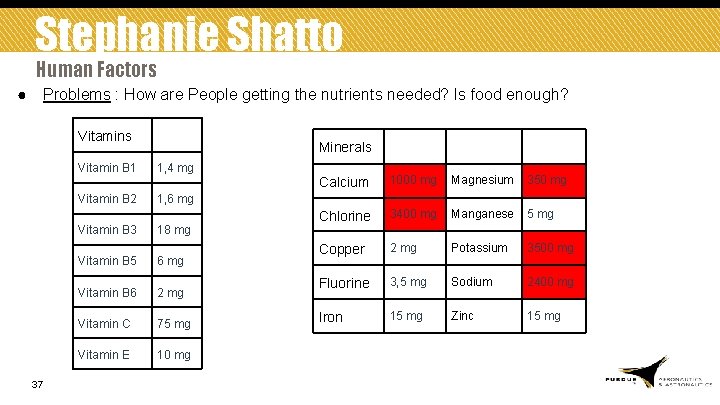
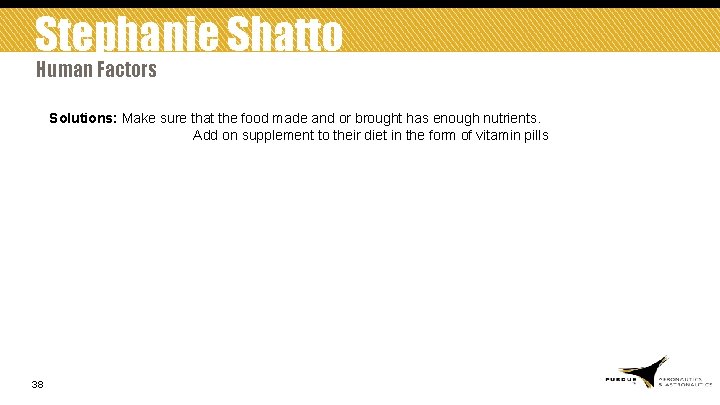
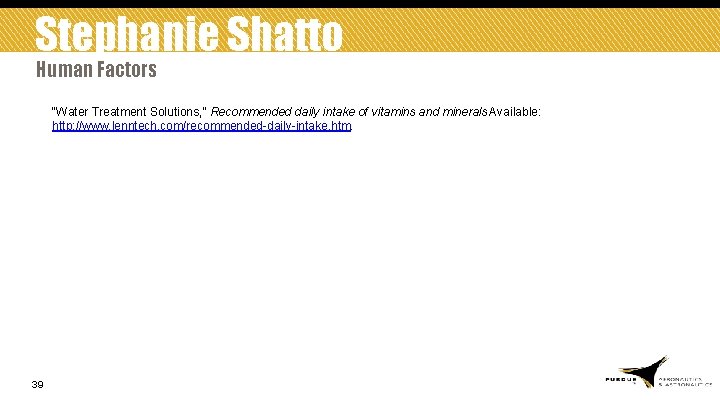
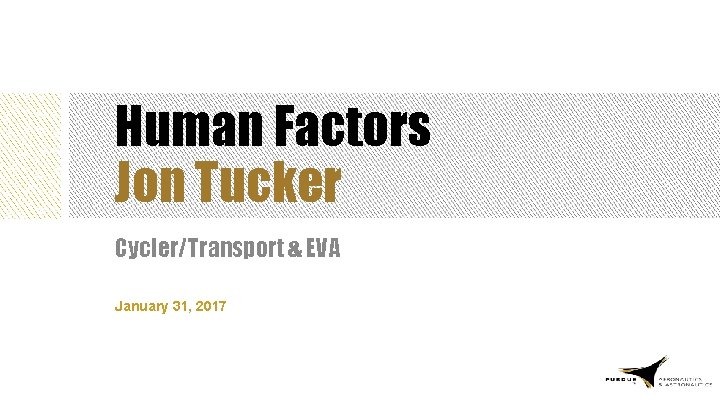
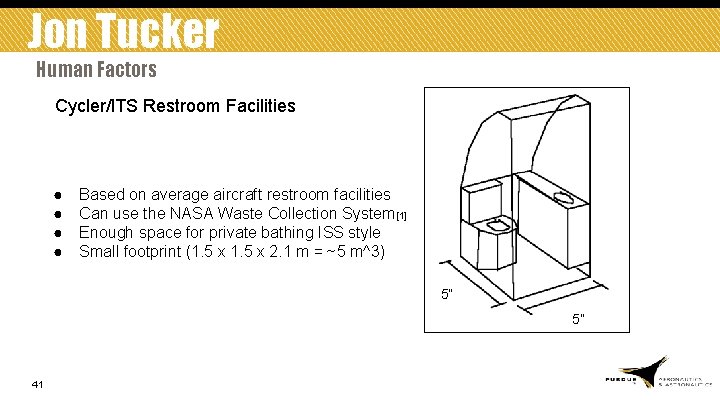
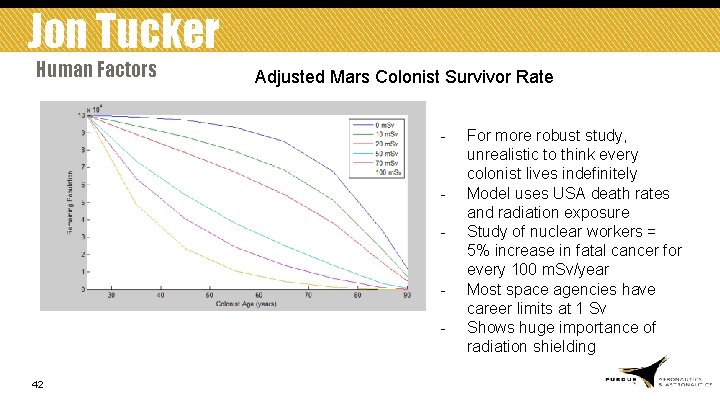
![Jon Tucker Human Factors References [1] “Waste Collection System, ” https: //spaceflight. nasa. gov/shuttle/reference//shutref/orbiter/eclss/wcs. Jon Tucker Human Factors References [1] “Waste Collection System, ” https: //spaceflight. nasa. gov/shuttle/reference//shutref/orbiter/eclss/wcs.](https://slidetodoc.com/presentation_image_h/184fd3da0ebff5691a469b53cfd00971/image-43.jpg)
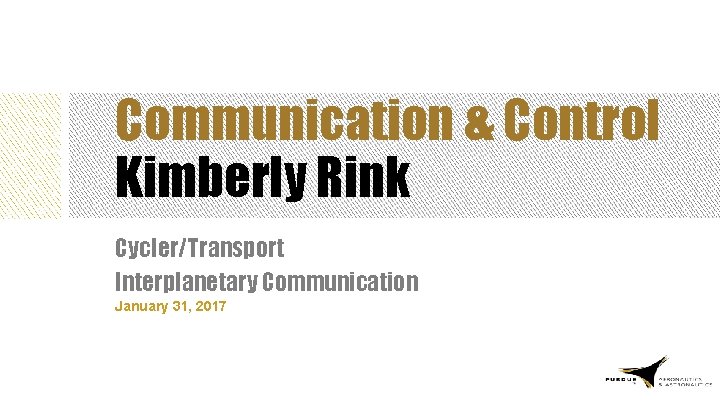
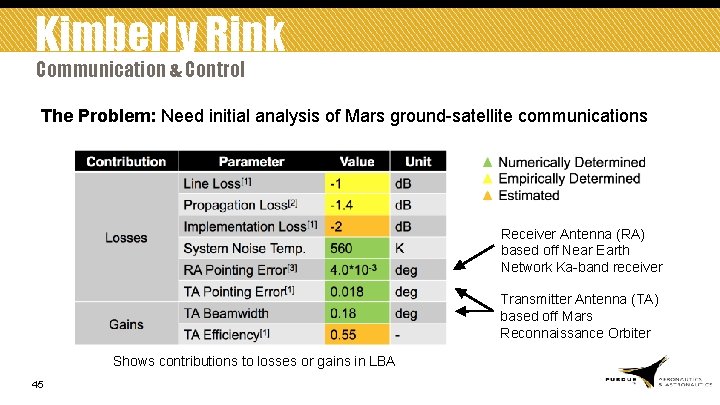
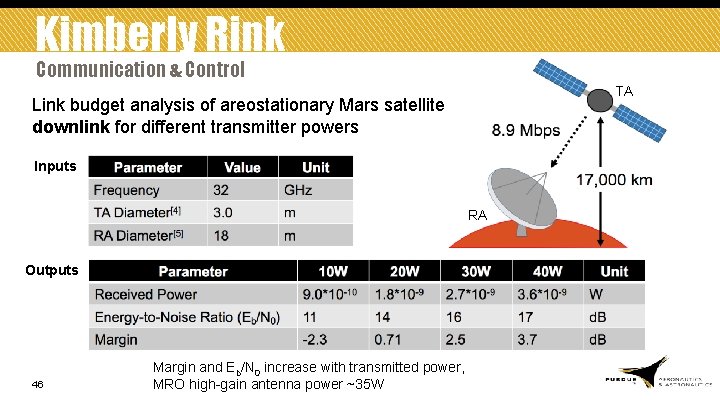
![Kimberly Rink Communication & Control Backup Slide References: [1] Larson, W. and Wertz, J. Kimberly Rink Communication & Control Backup Slide References: [1] Larson, W. and Wertz, J.](https://slidetodoc.com/presentation_image_h/184fd3da0ebff5691a469b53cfd00971/image-47.jpg)
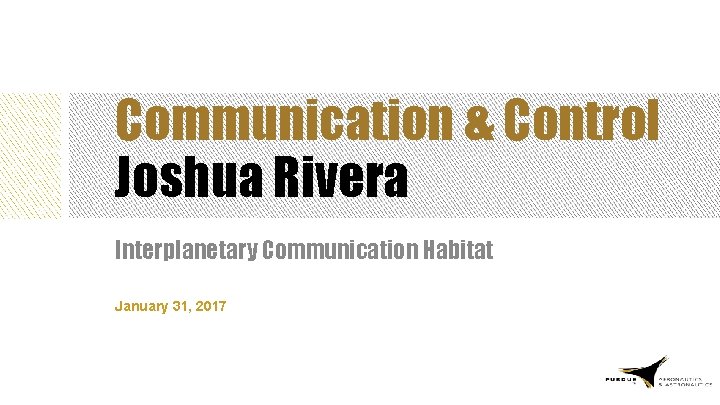
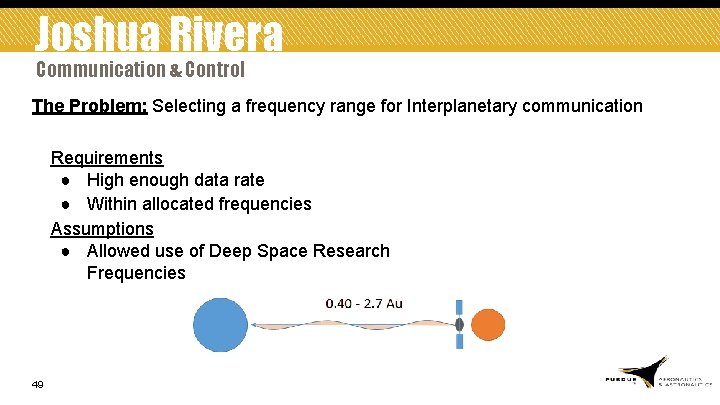
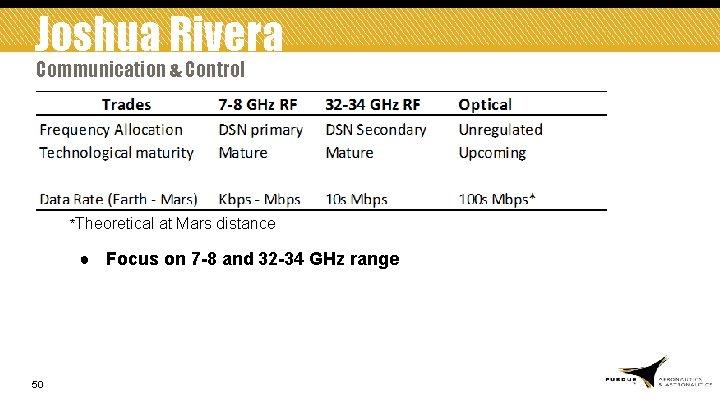
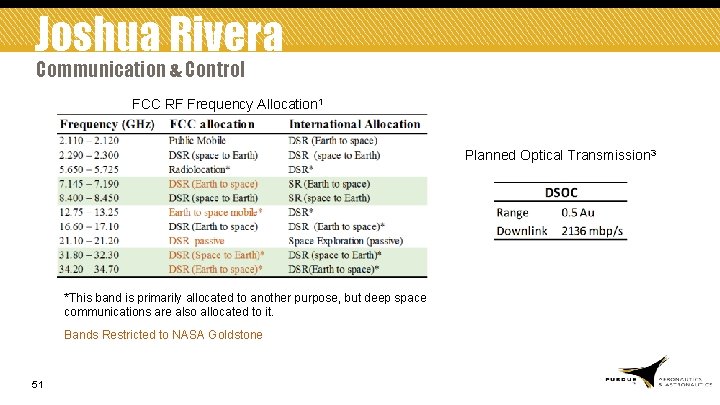
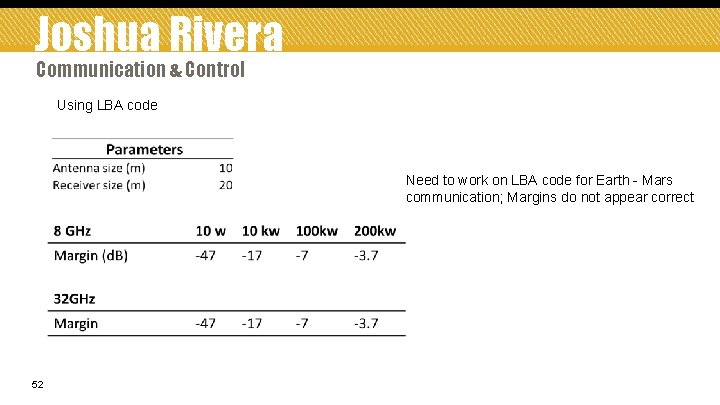
![Joshua Rivera Communication & Control References [1] “FCC Online Table of Frequency Allocations, ” Joshua Rivera Communication & Control References [1] “FCC Online Table of Frequency Allocations, ”](https://slidetodoc.com/presentation_image_h/184fd3da0ebff5691a469b53cfd00971/image-53.jpg)
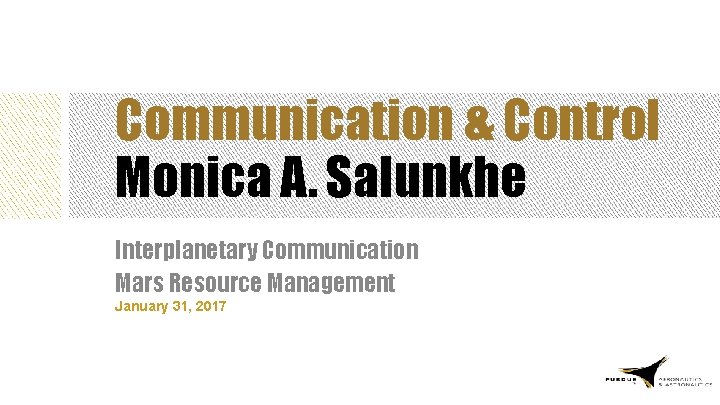
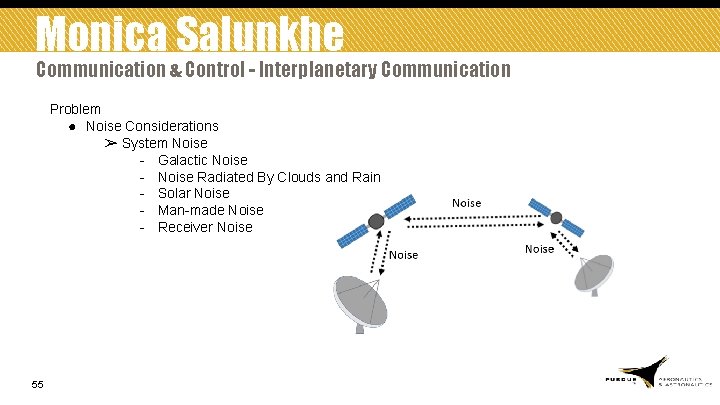
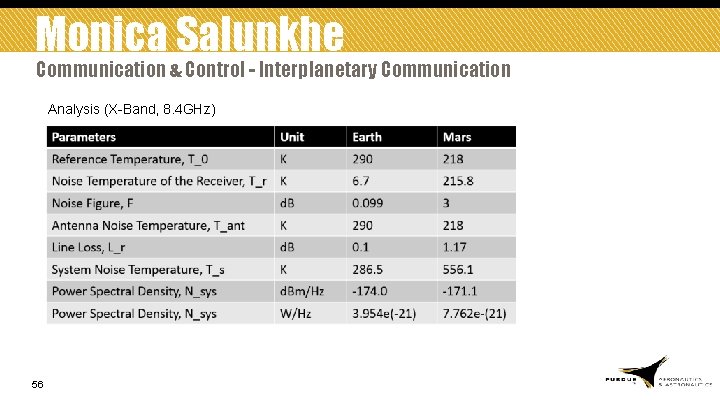
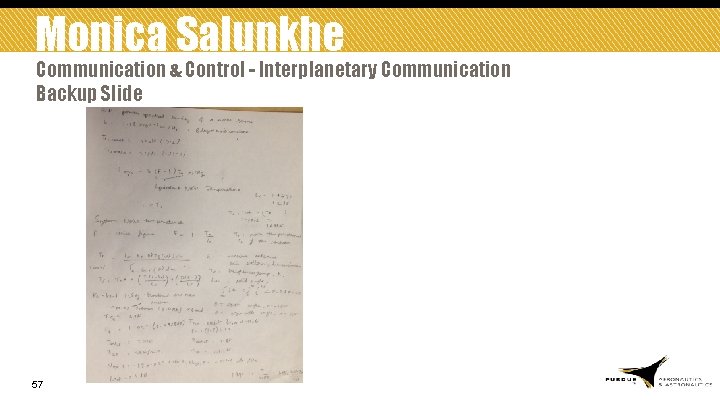
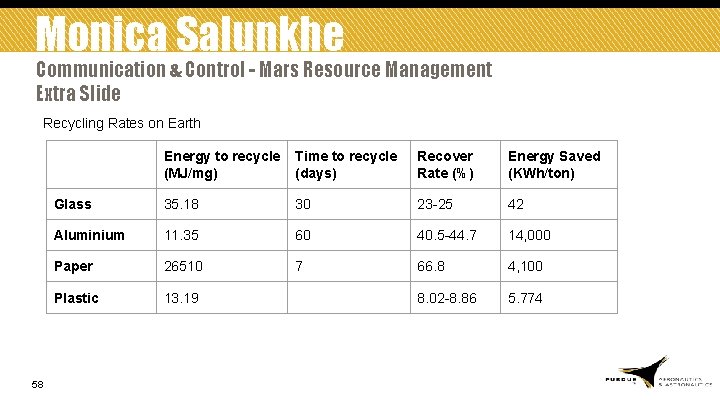
![Monica Salunkhe Communication & Control References [1] Larson, W. and Wertz, J. , “Communications Monica Salunkhe Communication & Control References [1] Larson, W. and Wertz, J. , “Communications](https://slidetodoc.com/presentation_image_h/184fd3da0ebff5691a469b53cfd00971/image-59.jpg)
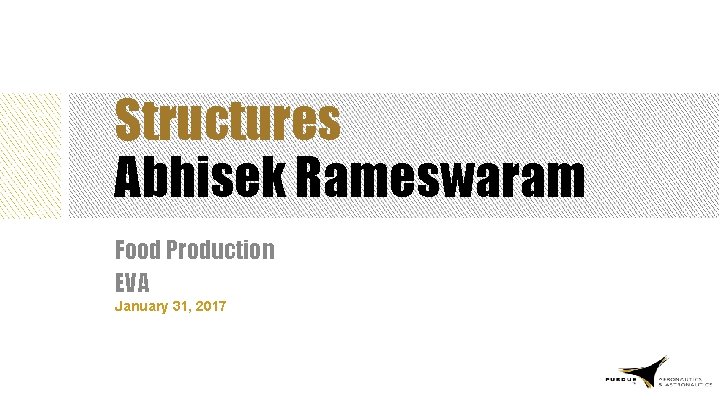
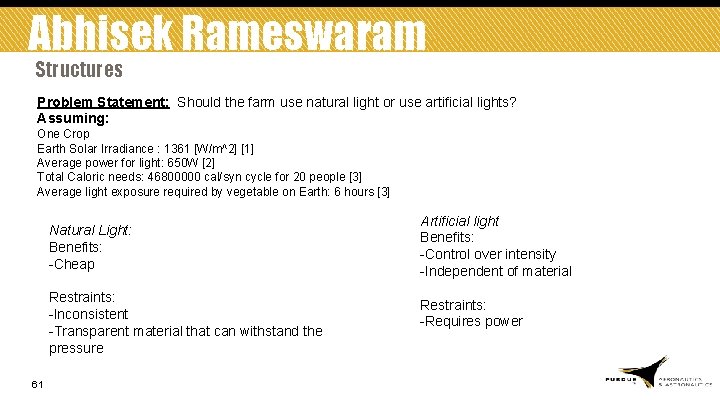
![Abhisek Rameswaram Structures Table 1: Size Requirements [3] Food Cal/m^2 Farm Size [m^2] Potatos Abhisek Rameswaram Structures Table 1: Size Requirements [3] Food Cal/m^2 Farm Size [m^2] Potatos](https://slidetodoc.com/presentation_image_h/184fd3da0ebff5691a469b53cfd00971/image-62.jpg)
![Abhisek Rameswaram Structures Back. Up References: [1]NASA. , “Mars Fact Sheet, ” Available: http: Abhisek Rameswaram Structures Back. Up References: [1]NASA. , “Mars Fact Sheet, ” Available: http:](https://slidetodoc.com/presentation_image_h/184fd3da0ebff5691a469b53cfd00971/image-63.jpg)
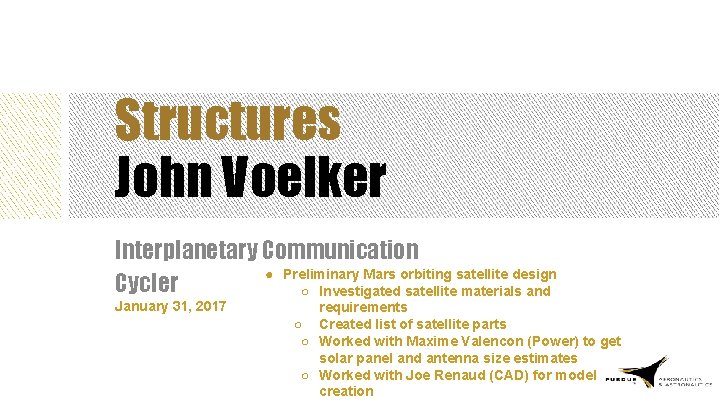
![John Voelker Structures & Interplanetary Communication Material Mass/Area [g/cm^2] Layup Position Beta Cloth 0. John Voelker Structures & Interplanetary Communication Material Mass/Area [g/cm^2] Layup Position Beta Cloth 0.](https://slidetodoc.com/presentation_image_h/184fd3da0ebff5691a469b53cfd00971/image-65.jpg)
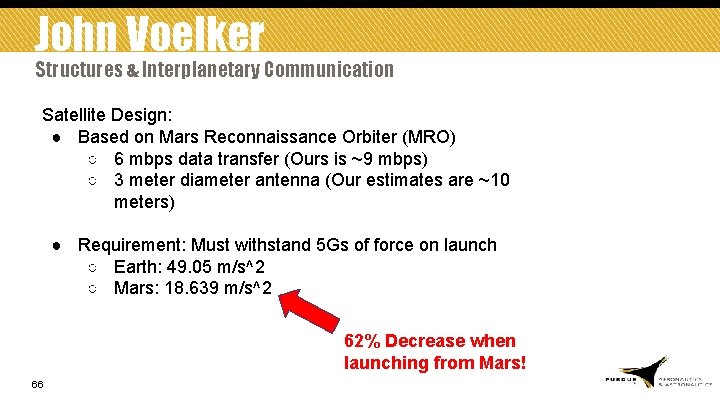
![John Voelker Structures & Interplanetary Communication References [1] Dooling, D. , and Finckenor, M. John Voelker Structures & Interplanetary Communication References [1] Dooling, D. , and Finckenor, M.](https://slidetodoc.com/presentation_image_h/184fd3da0ebff5691a469b53cfd00971/image-67.jpg)
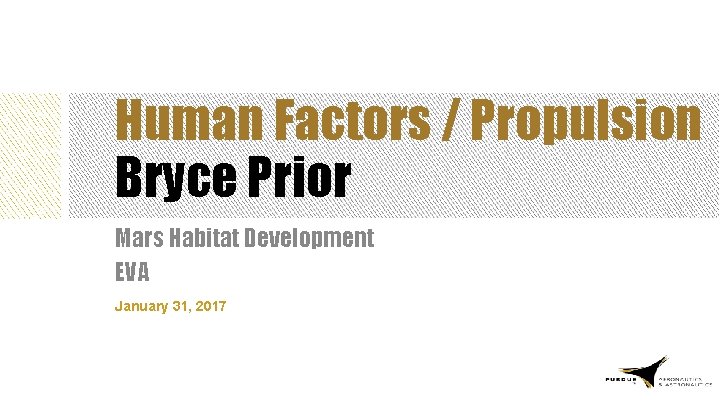
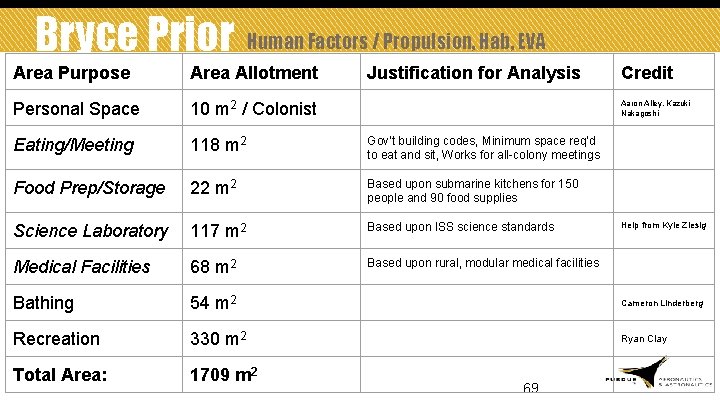
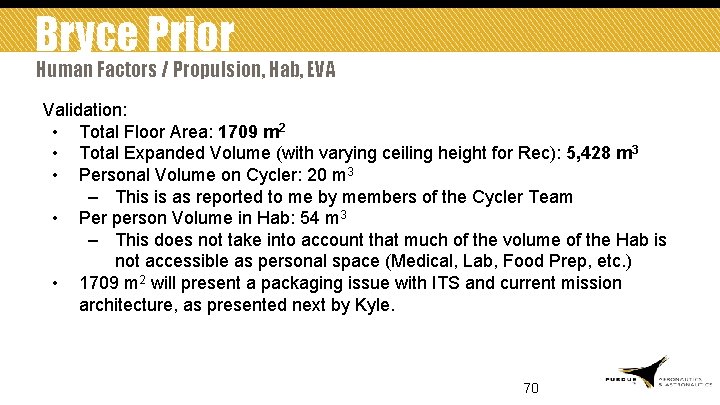
![Bryce Prior Human Factors / Propulsion, Hab, EVA [1] Shimabukuro, B. , “From the Bryce Prior Human Factors / Propulsion, Hab, EVA [1] Shimabukuro, B. , “From the](https://slidetodoc.com/presentation_image_h/184fd3da0ebff5691a469b53cfd00971/image-71.jpg)
![Bryce Prior Human Factors / Propulsion, Hab, EVA [15] New York city government “Chapter Bryce Prior Human Factors / Propulsion, Hab, EVA [15] New York city government “Chapter](https://slidetodoc.com/presentation_image_h/184fd3da0ebff5691a469b53cfd00971/image-72.jpg)
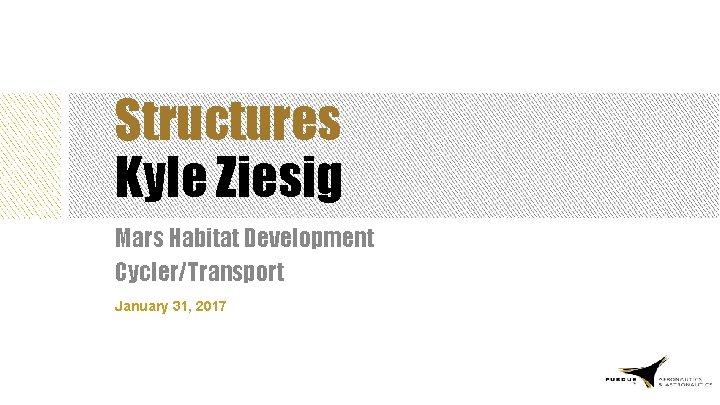
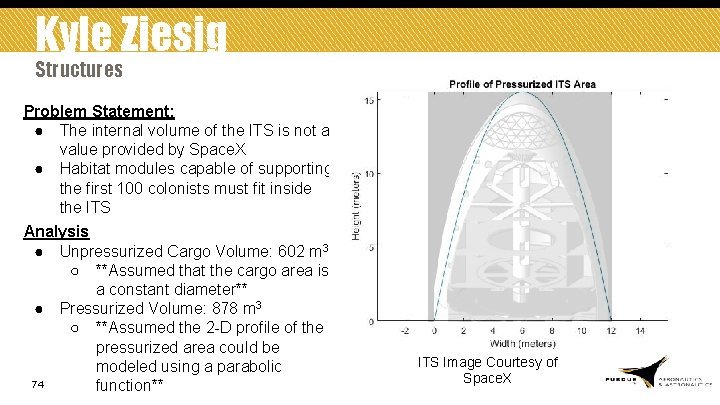
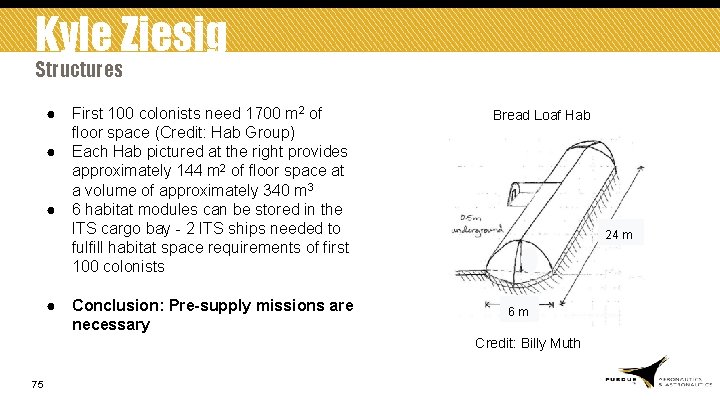
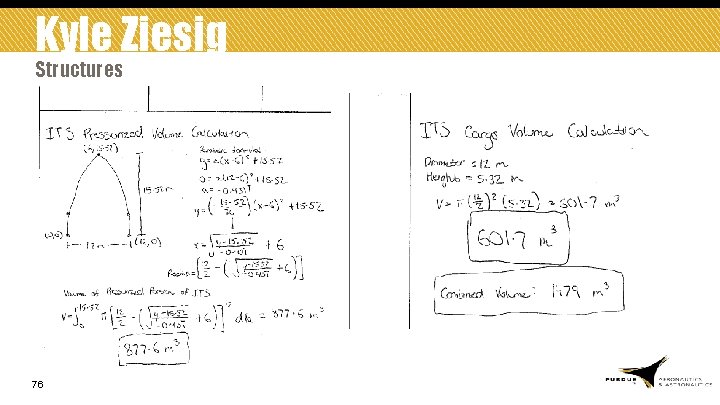
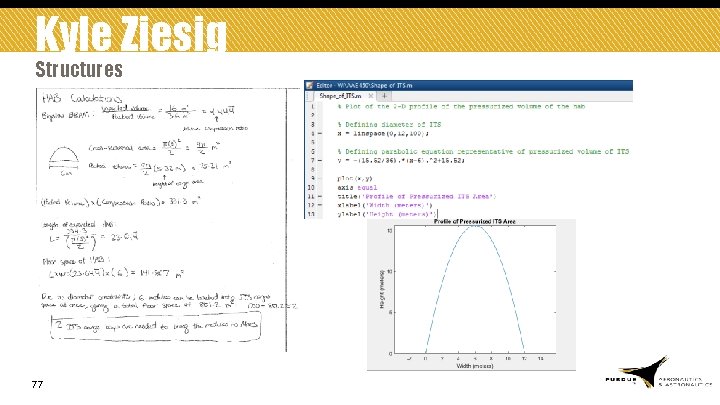
![Kyle Ziesig Structures References [1] “Space. X, ” Space. X Available: http: //www. spacex. Kyle Ziesig Structures References [1] “Space. X, ” Space. X Available: http: //www. spacex.](https://slidetodoc.com/presentation_image_h/184fd3da0ebff5691a469b53cfd00971/image-78.jpg)
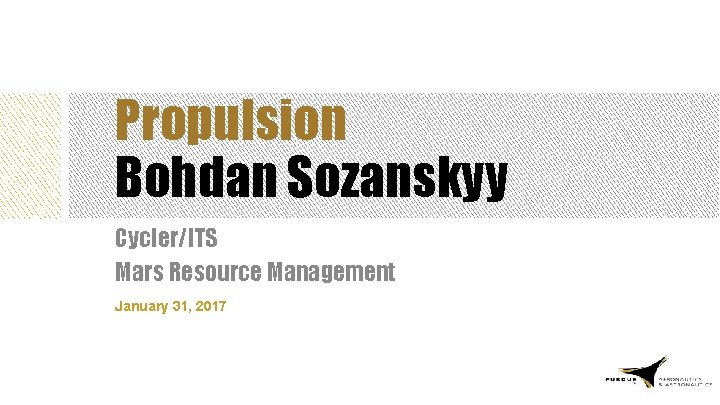
![Bohdan Sozanksyy Propulsion */ Problem: Producing fuel for return trip (LOX/Methane) ITS Propellant [1]: Bohdan Sozanksyy Propulsion */ Problem: Producing fuel for return trip (LOX/Methane) ITS Propellant [1]:](https://slidetodoc.com/presentation_image_h/184fd3da0ebff5691a469b53cfd00971/image-80.jpg)
![Bohdan Sozanksyy Propulsion */ Reactor Unit Size Estimate [2] ● Producing 500 kg/day of Bohdan Sozanksyy Propulsion */ Reactor Unit Size Estimate [2] ● Producing 500 kg/day of](https://slidetodoc.com/presentation_image_h/184fd3da0ebff5691a469b53cfd00971/image-81.jpg)
![Bohdan Sozanksyy Propulsion */ References [1] Richardson, D. , “Elon Musk shows off Interplanetary Bohdan Sozanksyy Propulsion */ References [1] Richardson, D. , “Elon Musk shows off Interplanetary](https://slidetodoc.com/presentation_image_h/184fd3da0ebff5691a469b53cfd00971/image-82.jpg)
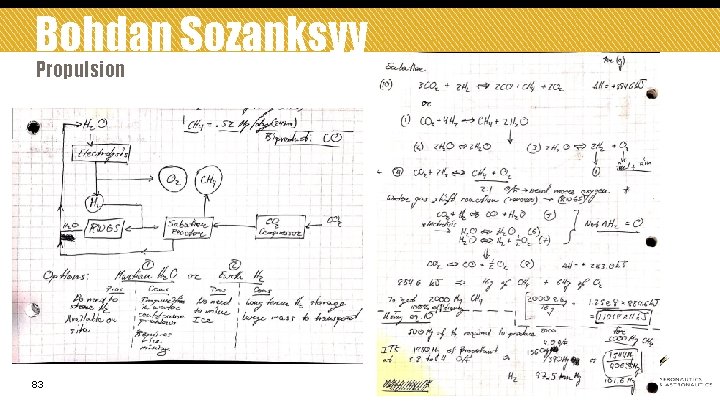
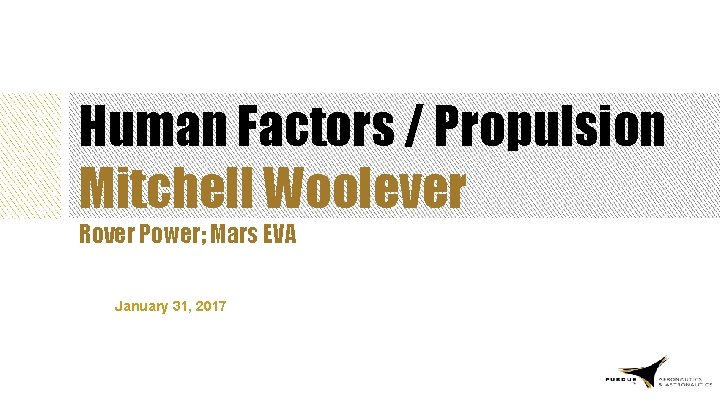
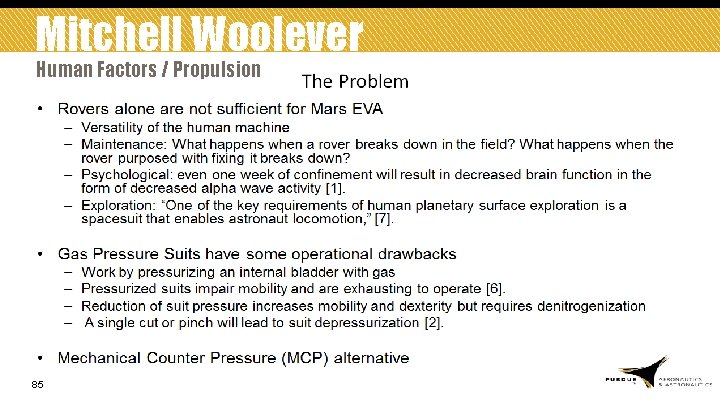
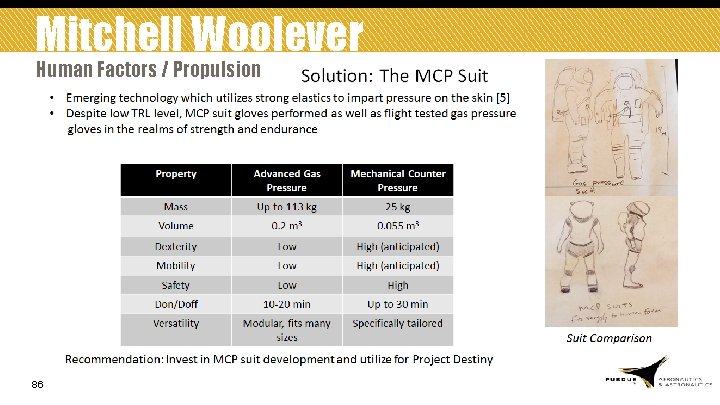
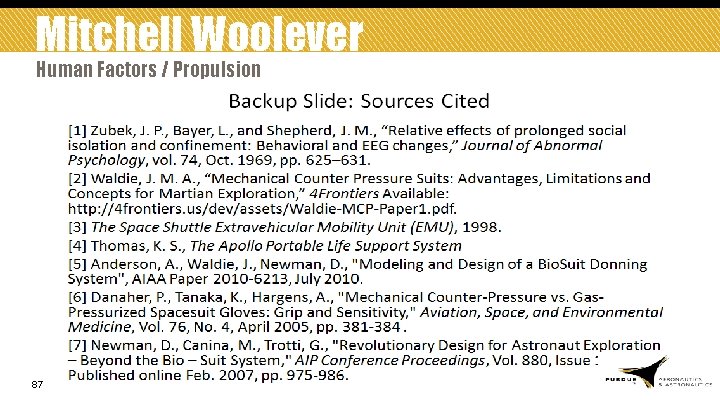
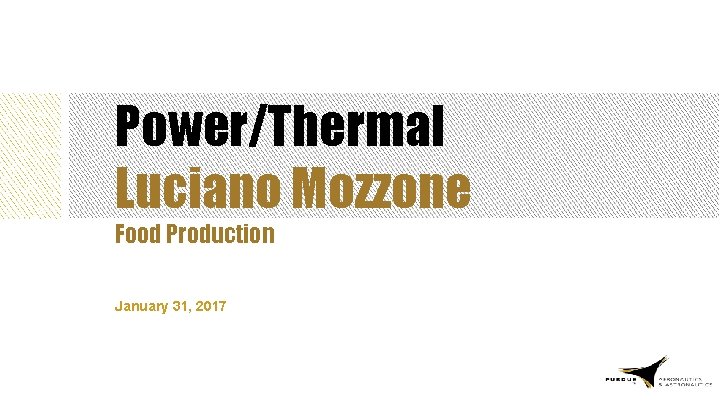
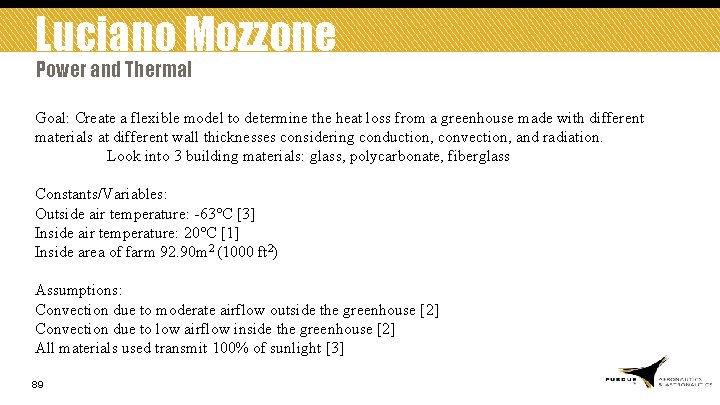
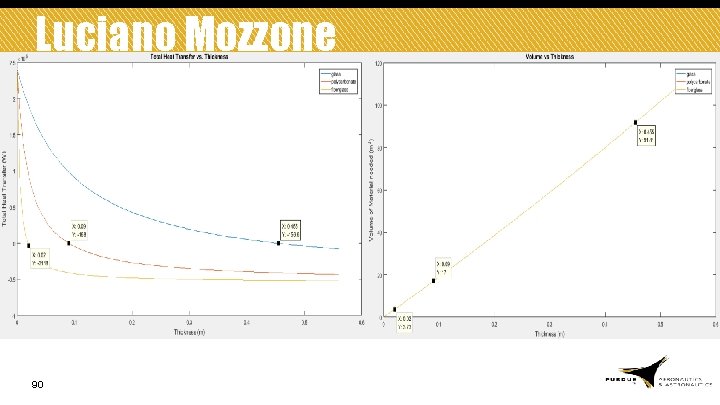
![Luciano Mozzone Power and Thermal Backup Slide: Resources [1] Iga, J. L. , Iga, Luciano Mozzone Power and Thermal Backup Slide: Resources [1] Iga, J. L. , Iga,](https://slidetodoc.com/presentation_image_h/184fd3da0ebff5691a469b53cfd00971/image-91.jpg)
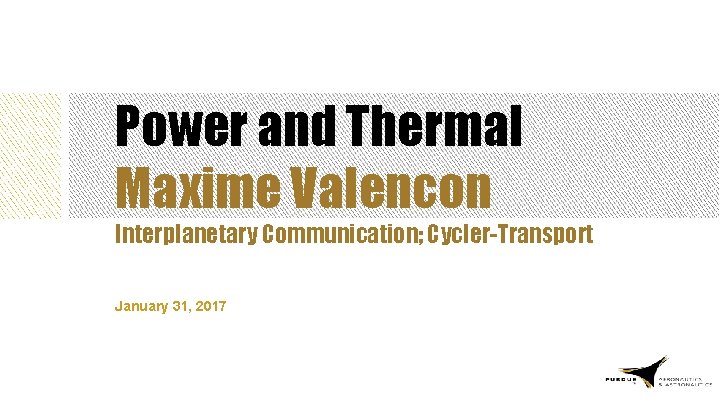
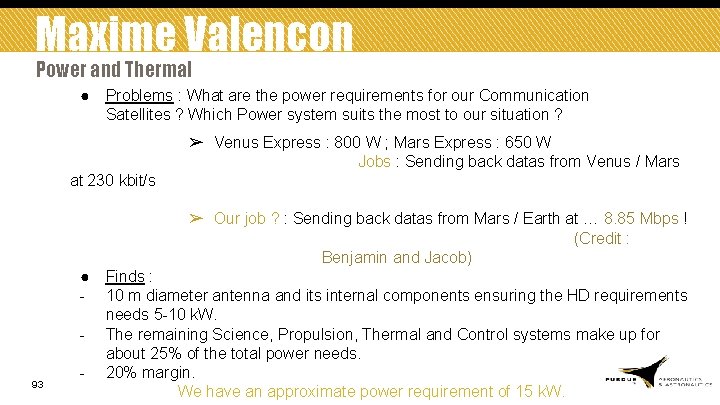
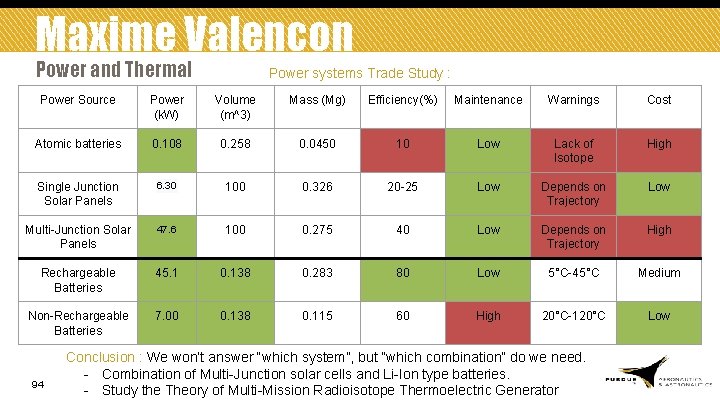
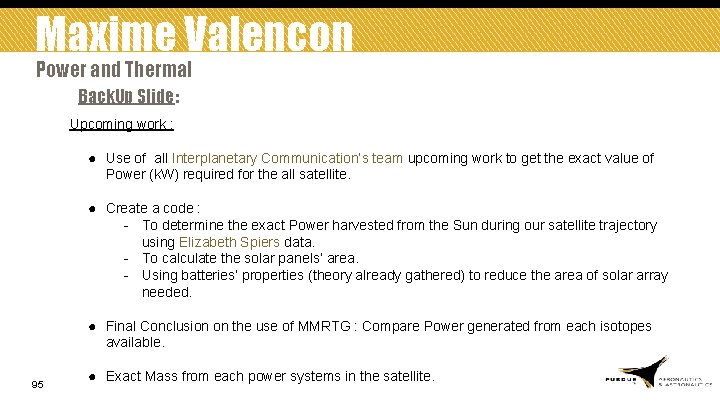
![Maxime Valencon Power and Thermal References: 96 [1] J. Arenberg, J. Flynn , A. Maxime Valencon Power and Thermal References: 96 [1] J. Arenberg, J. Flynn , A.](https://slidetodoc.com/presentation_image_h/184fd3da0ebff5691a469b53cfd00971/image-96.jpg)
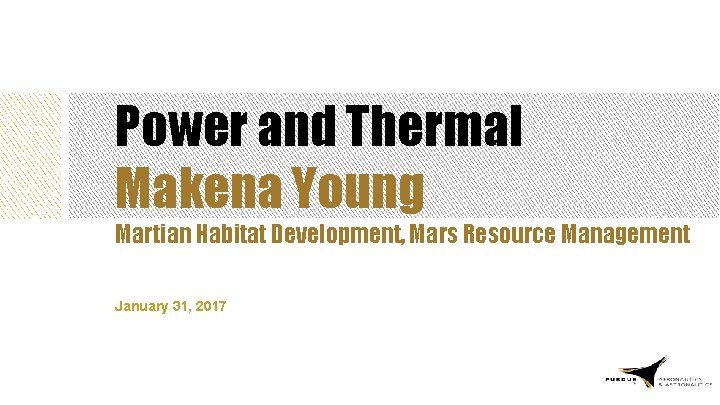
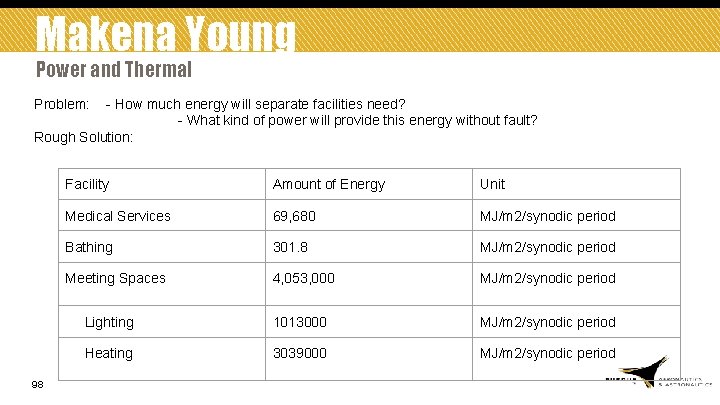
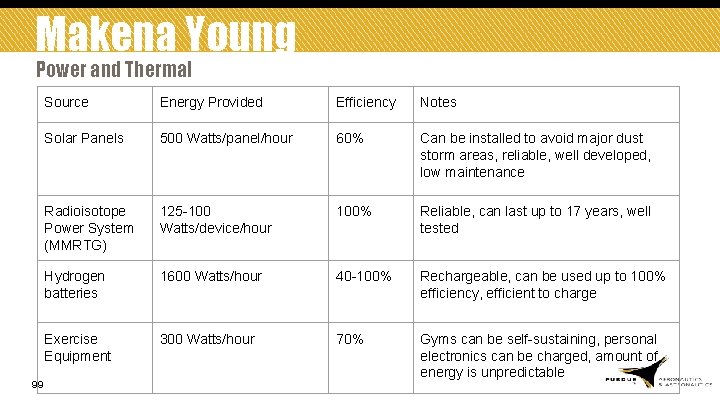
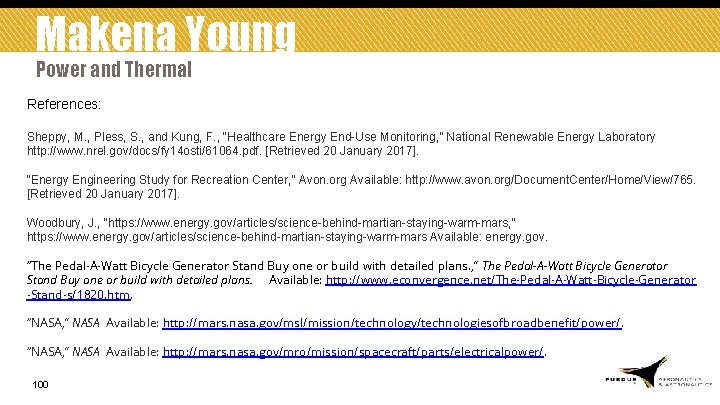
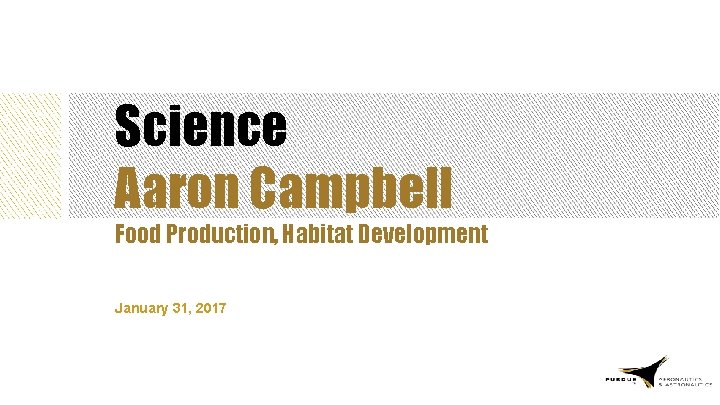
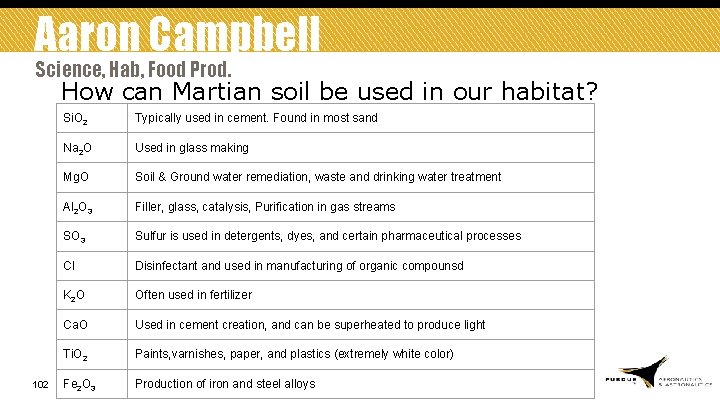
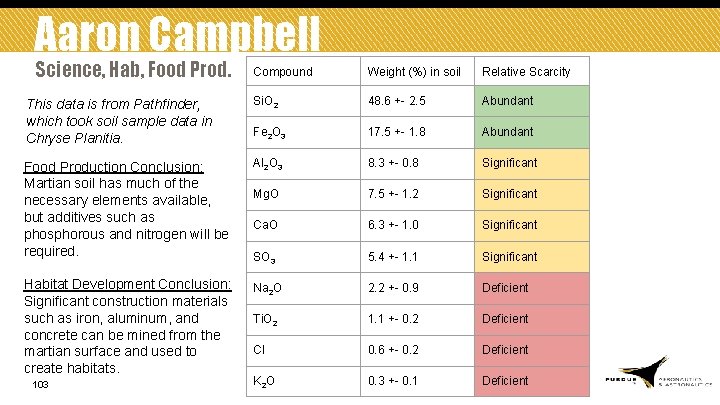
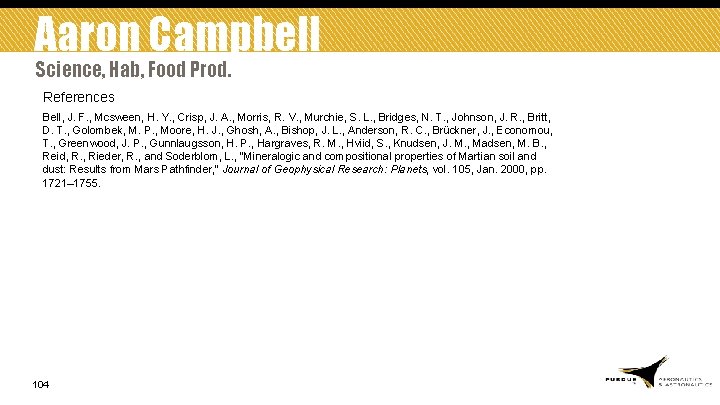
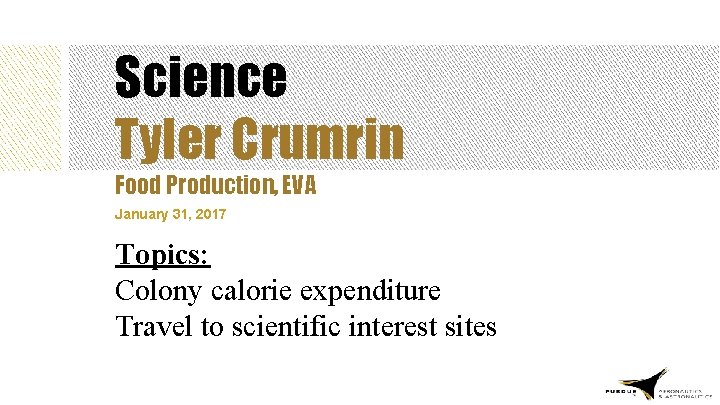
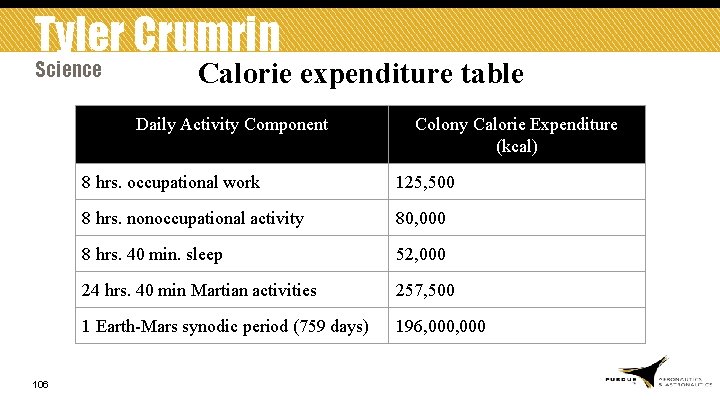
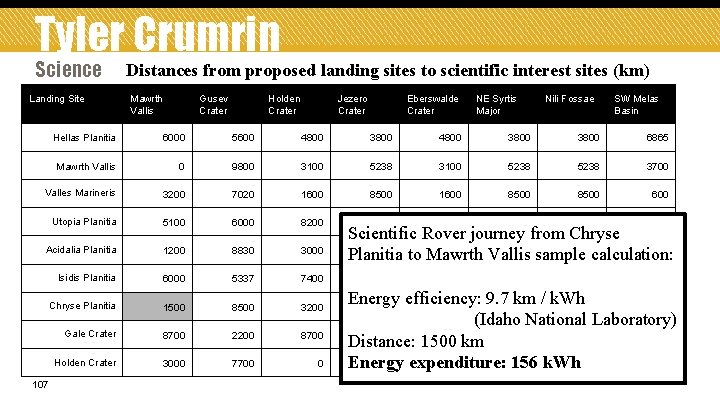
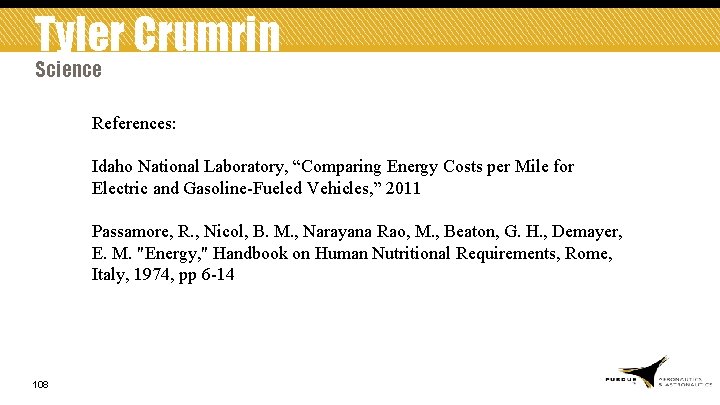
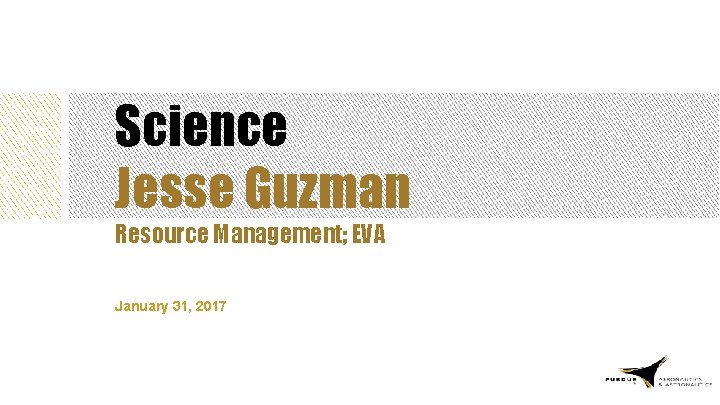
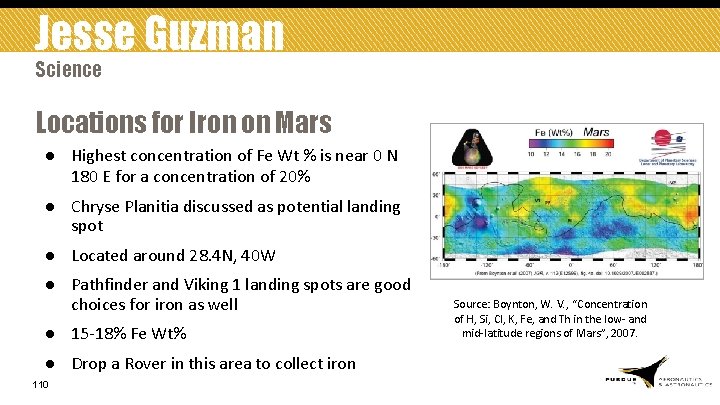
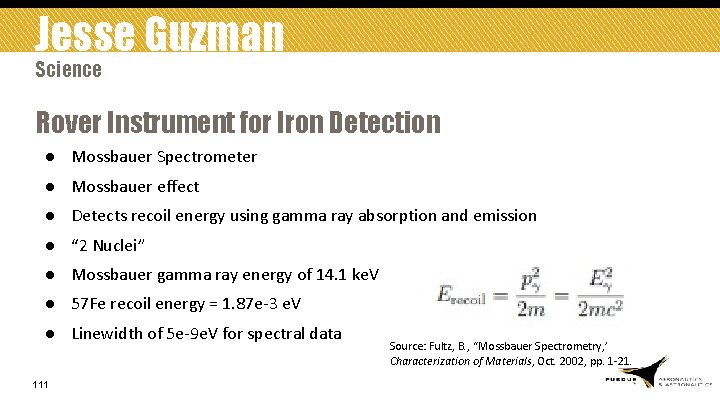
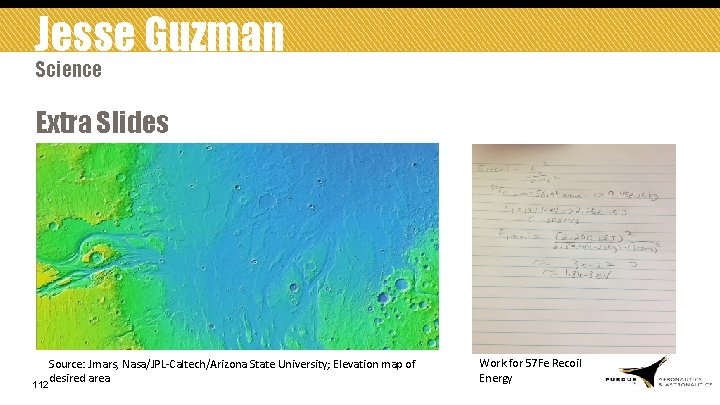
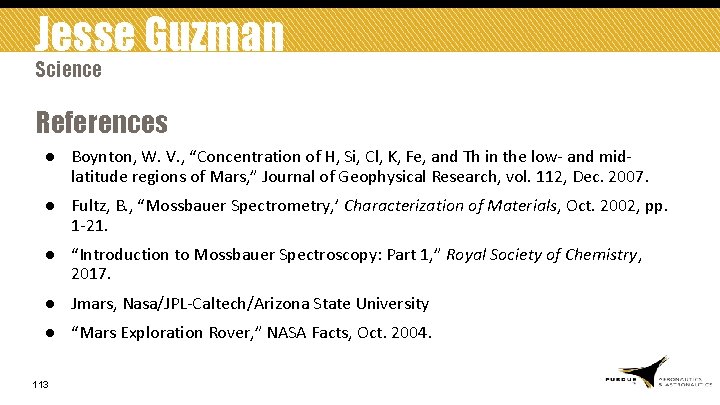
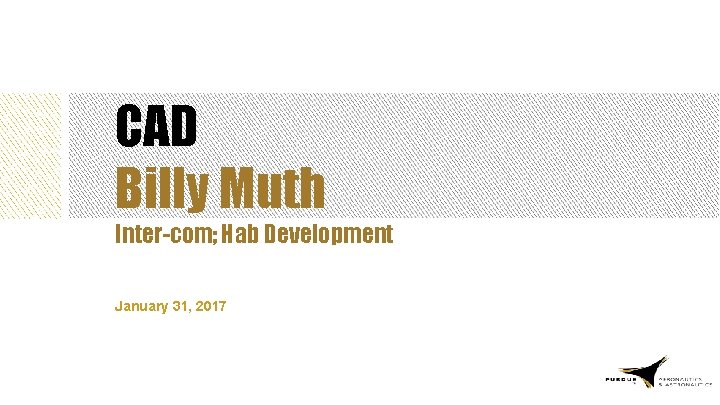
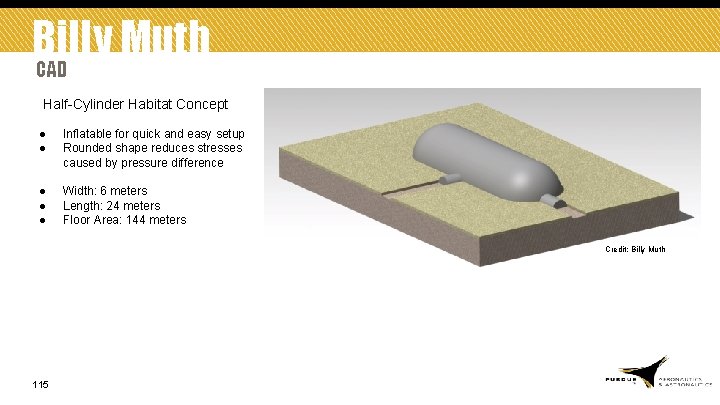
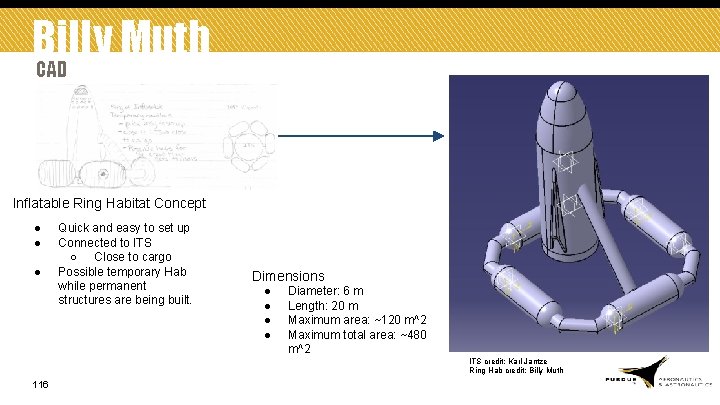
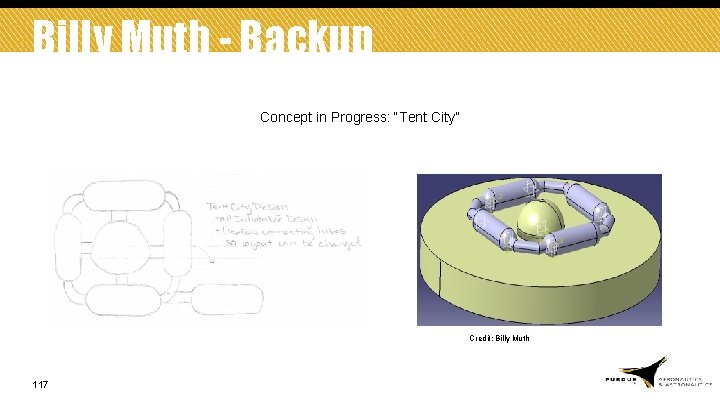
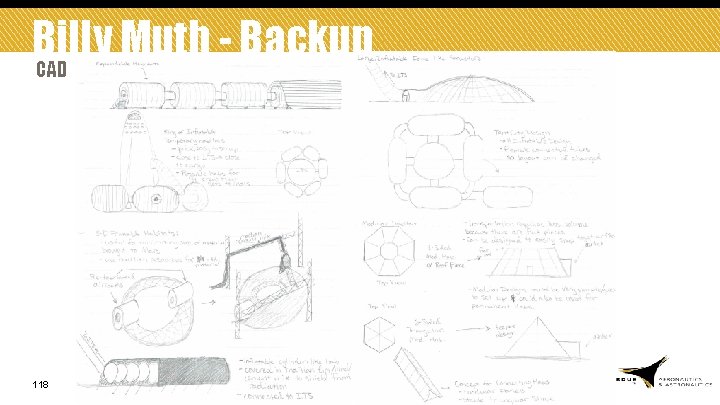
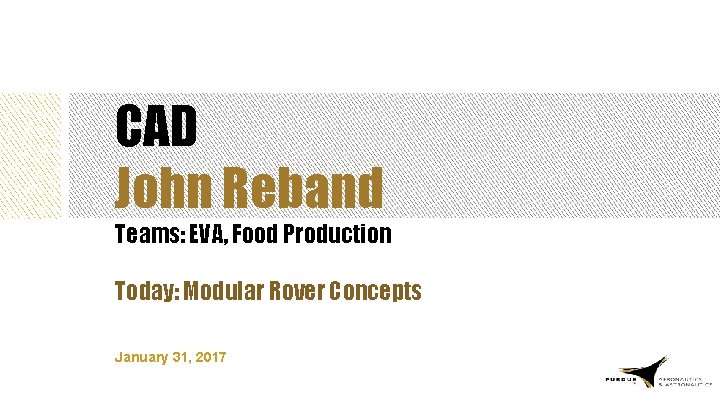
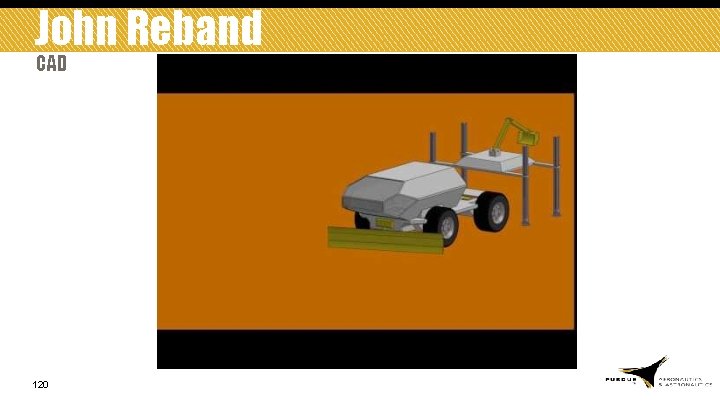
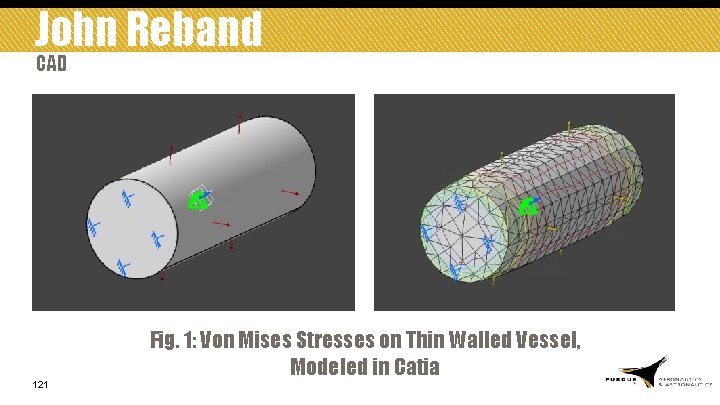
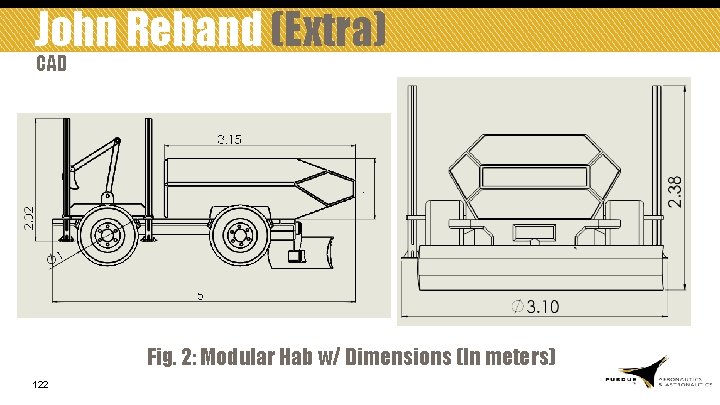
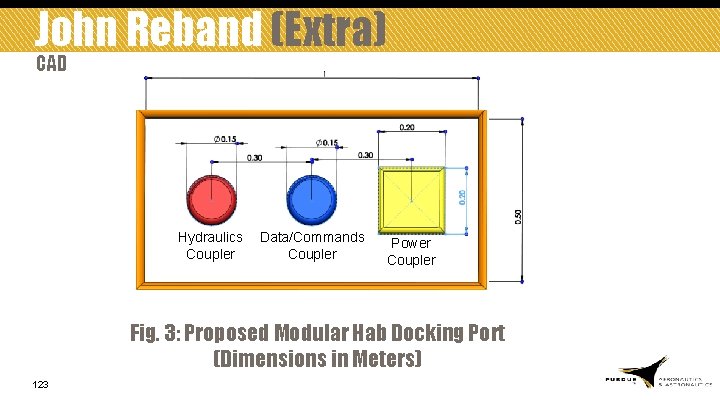
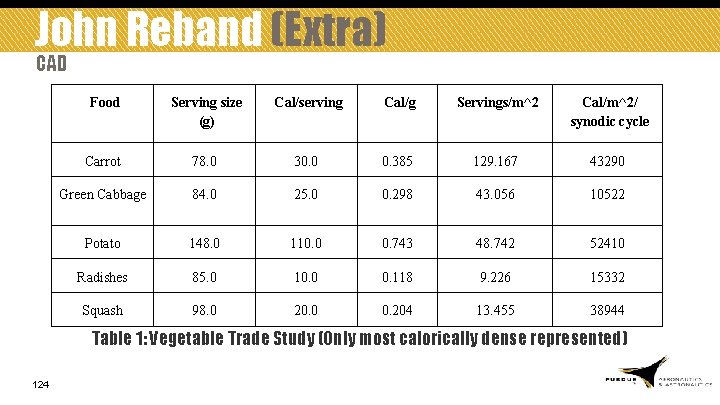
![John Reband (Extra) CAD References [1] “Vegetable Nutrition Facts, ” fda. gov Available: http: John Reband (Extra) CAD References [1] “Vegetable Nutrition Facts, ” fda. gov Available: http:](https://slidetodoc.com/presentation_image_h/184fd3da0ebff5691a469b53cfd00971/image-125.jpg)
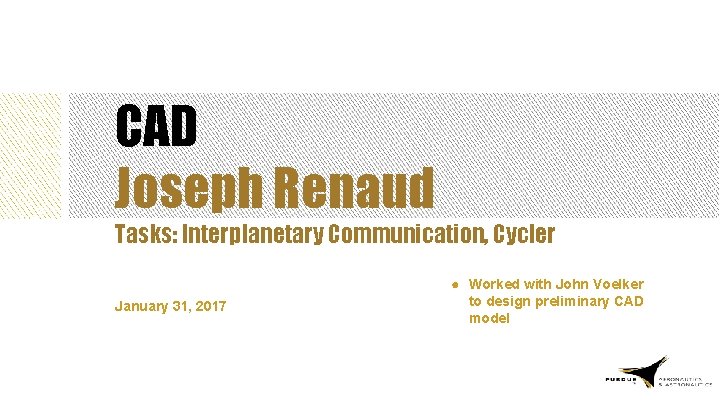
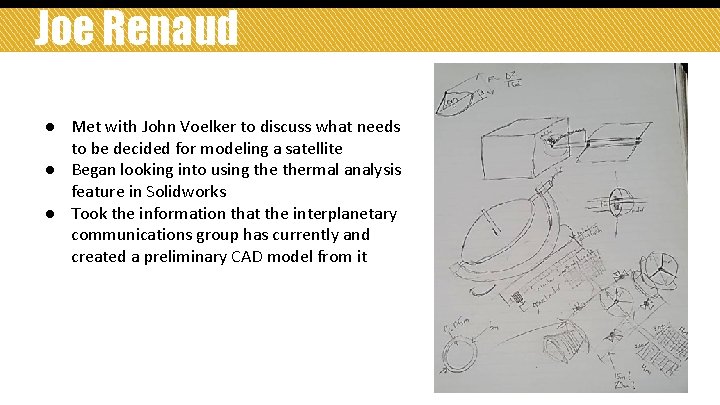
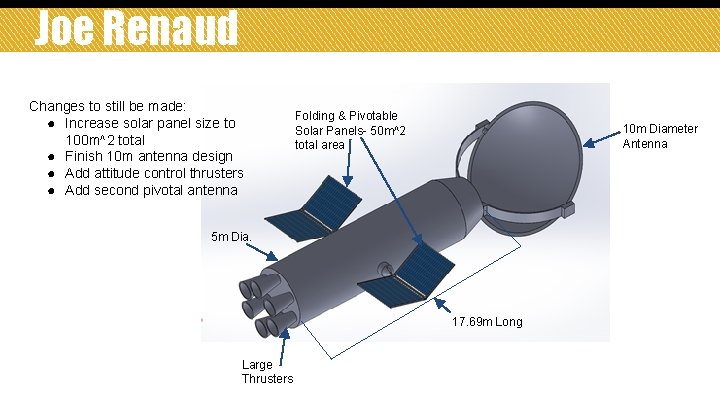
- Slides: 128
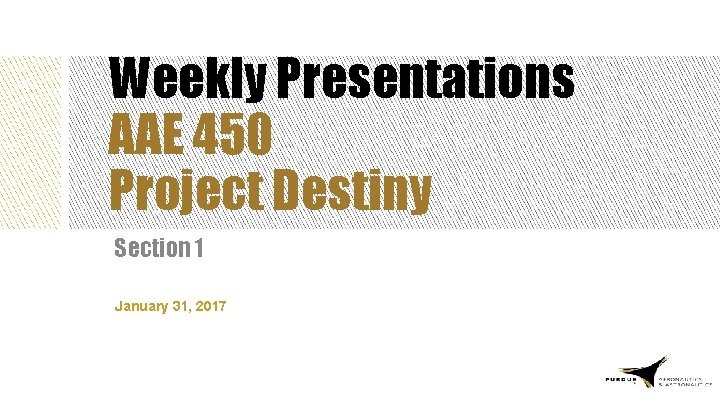
Weekly Presentations AAE 450 Project Destiny Section 1 January 31, 2017
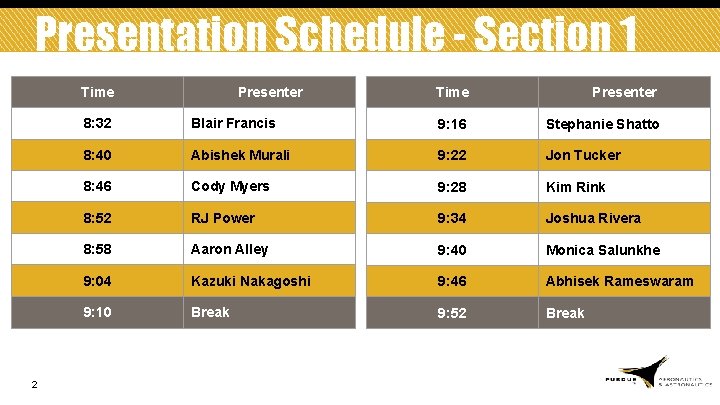
Presentation Schedule - Section 1 Time 2 Presenter Time Presenter 8: 32 Blair Francis 9: 16 Stephanie Shatto 8: 40 Abishek Murali 9: 22 Jon Tucker 8: 46 Cody Myers 9: 28 Kim Rink 8: 52 RJ Power 9: 34 Joshua Rivera 8: 58 Aaron Alley 9: 40 Monica Salunkhe 9: 04 Kazuki Nakagoshi 9: 46 Abhisek Rameswaram 9: 10 Break 9: 52 Break
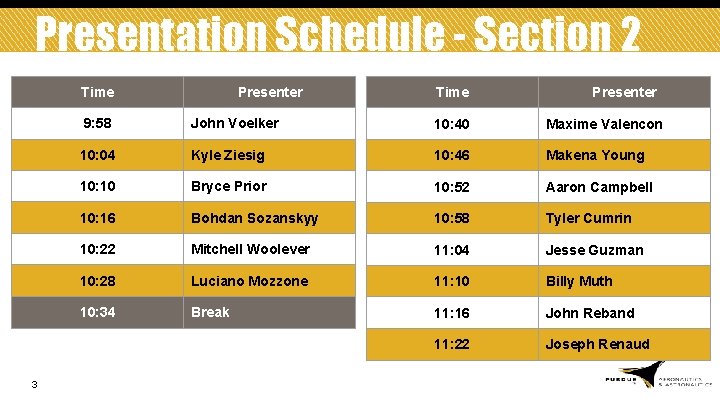
Presentation Schedule - Section 2 Time 3 Presenter Time Presenter 9: 58 John Voelker 10: 40 Maxime Valencon 10: 04 Kyle Ziesig 10: 46 Makena Young 10: 10 Bryce Prior 10: 52 Aaron Campbell 10: 16 Bohdan Sozanskyy 10: 58 Tyler Cumrin 10: 22 Mitchell Woolever 11: 04 Jesse Guzman 10: 28 Luciano Mozzone 11: 10 Billy Muth 10: 34 Break 11: 16 John Reband 11: 22 Joseph Renaud
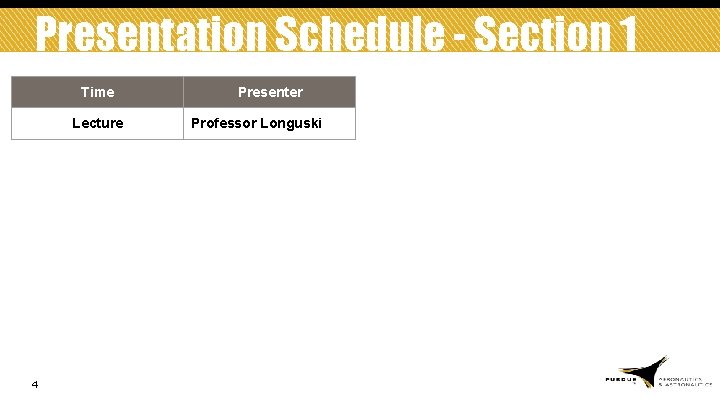
Presentation Schedule - Section 1 Time Lecture 4 Presenter Professor Longuski
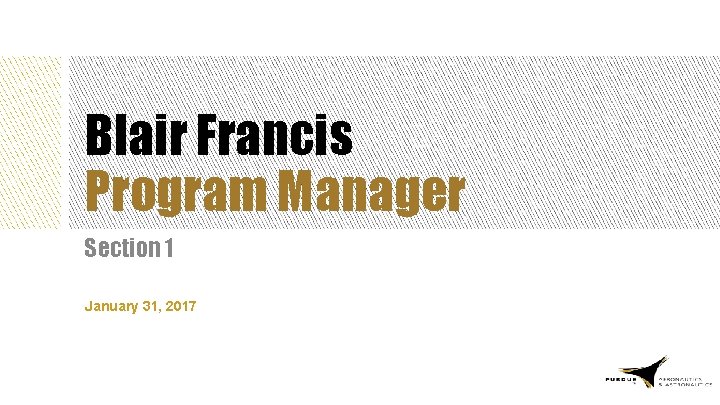
Blair Francis Program Manager Section 1 January 31, 2017
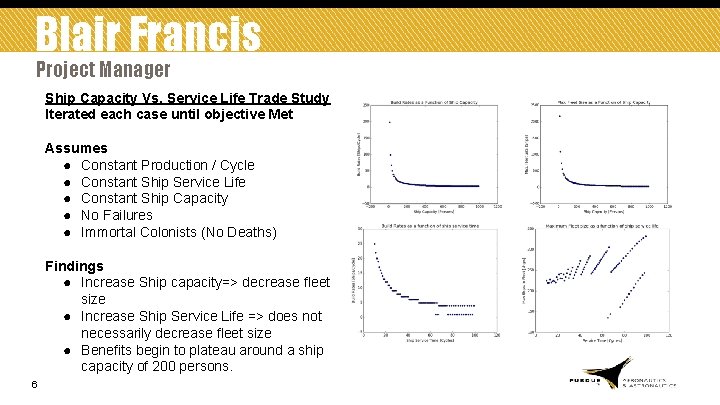
Blair Francis Project Manager Ship Capacity Vs. Service Life Trade Study Iterated each case until objective Met Assumes ● Constant Production / Cycle ● Constant Ship Service Life ● Constant Ship Capacity ● No Failures ● Immortal Colonists (No Deaths) Findings ● Increase Ship capacity=> decrease fleet size ● Increase Ship Service Life => does not necessarily decrease fleet size ● Benefits begin to plateau around a ship capacity of 200 persons. 6
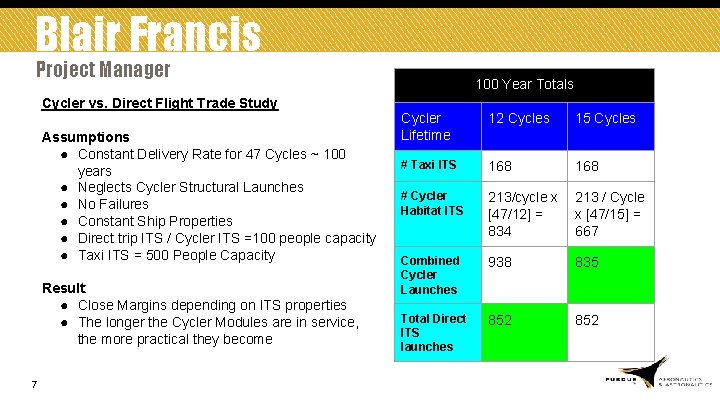
Blair Francis Project Manager Cycler vs. Direct Flight Trade Study Assumptions ● Constant Delivery Rate for 47 Cycles ~ 100 years ● Neglects Cycler Structural Launches ● No Failures ● Constant Ship Properties ● Direct trip ITS / Cycler ITS =100 people capacity ● Taxi ITS = 500 People Capacity Result: ● Close Margins depending on ITS properties ● The longer the Cycler Modules are in service, the more practical they become 7 100 Year Totals Cycler Lifetime 12 Cycles 15 Cycles # Taxi ITS 168 # Cycler Habitat ITS 213/cycle x [47/12] = 834 213 / Cycle x [47/15] = 667 Combined Cycler Launches 938 835 Total Direct ITS launches 852
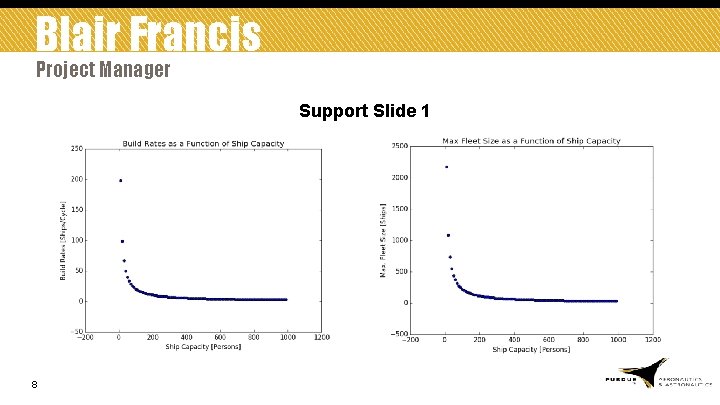
Blair Francis Project Manager Support Slide 1 8
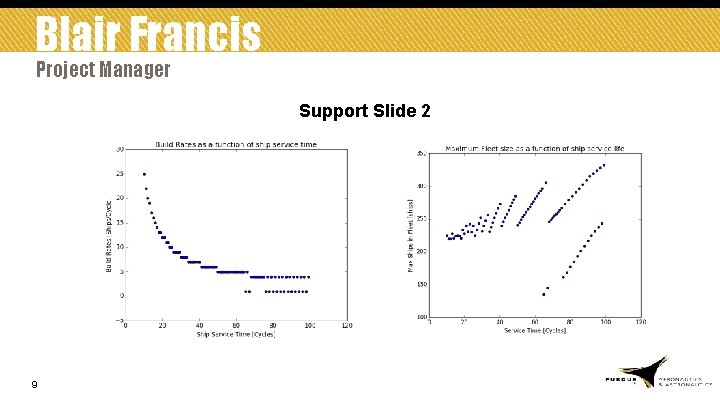
Blair Francis Project Manager Support Slide 2 9
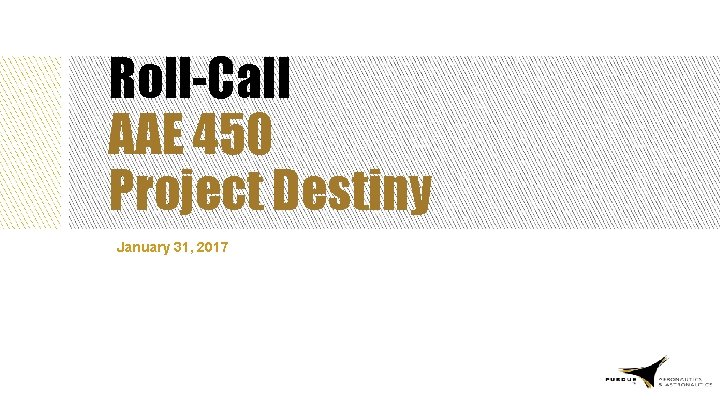
Roll-Call AAE 450 Project Destiny January 31, 2017
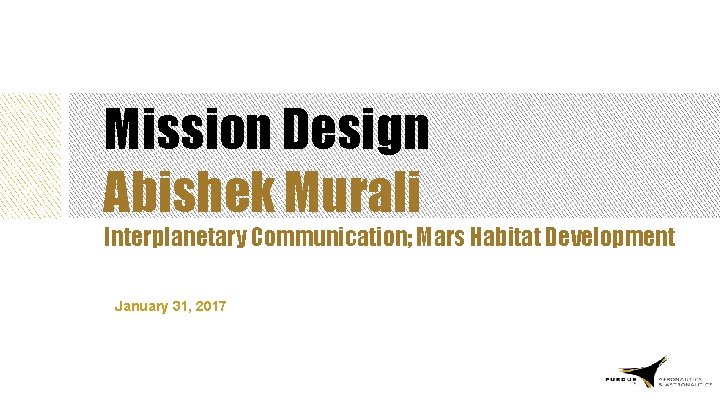
Mission Design Abishek Murali Interplanetary Communication; Mars Habitat Development January 31, 2017
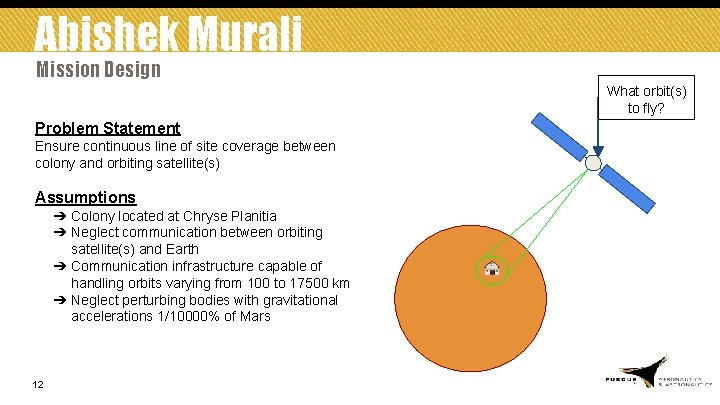
Abishek Murali Mission Design What orbit(s) to fly? Problem Statement Ensure continuous line of site coverage between colony and orbiting satellite(s) Assumptions ➔ Colony located at Chryse Planitia ➔ Neglect communication between orbiting satellite(s) and Earth ➔ Communication infrastructure capable of handling orbits varying from 100 to 17500 km ➔ Neglect perturbing bodies with gravitational accelerations 1/10000% of Mars 12
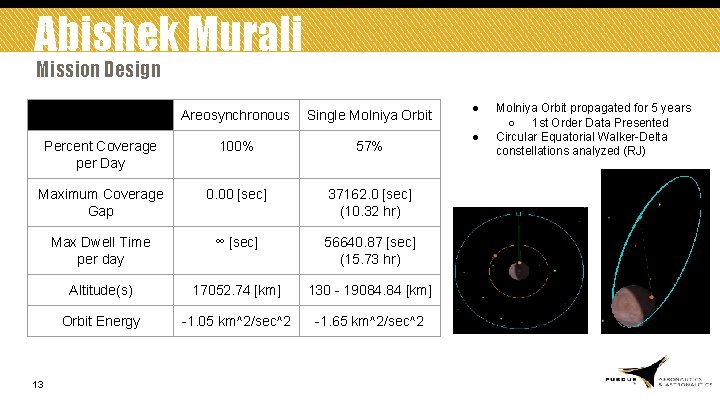
Abishek Murali Mission Design Areosynchronous Single Molniya Orbit Percent Coverage per Day 100% 57% Maximum Coverage Gap 0. 00 [sec] 37162. 0 [sec] (10. 32 hr) Max Dwell Time per day ∞ [sec] 56640. 87 [sec] (15. 73 hr) Altitude(s) 17052. 74 [km] 130 - 19084. 84 [km] Orbit Energy -1. 05 km^2/sec^2 -1. 65 km^2/sec^2 13 ● ● Molniya Orbit propagated for 5 years ○ 1 st Order Data Presented Circular Equatorial Walker-Delta constellations analyzed (RJ)
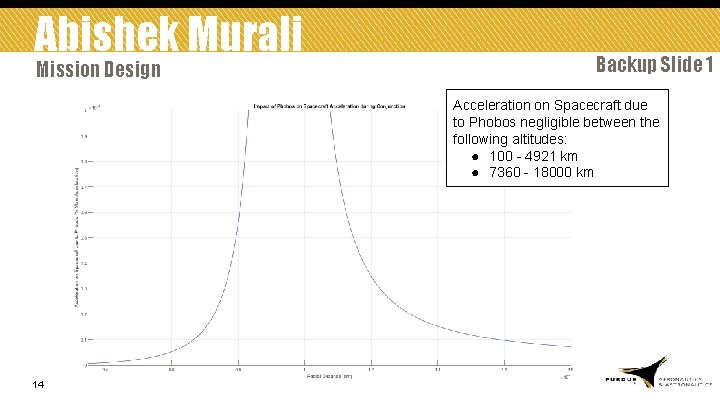
Abishek Murali Mission Design Backup Slide 1 Acceleration on Spacecraft due to Phobos negligible between the following altitudes: ● 100 - 4921 km ● 7360 - 18000 km 14
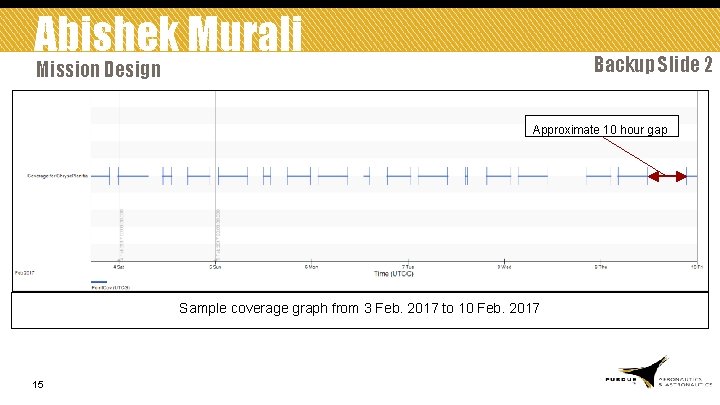
Abishek Murali Backup Slide 2 Mission Design Approximate 10 hour gap Sample coverage graph from 3 Feb. 2017 to 10 Feb. 2017 15
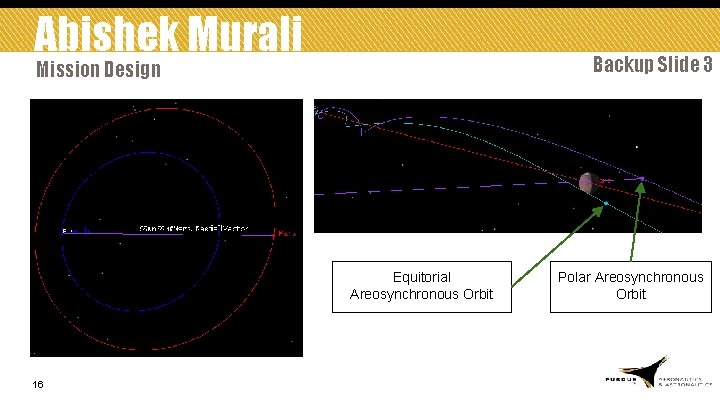
Abishek Murali Backup Slide 3 Mission Design Equitorial Areosynchronous Orbit 16 Polar Areosynchronous Orbit
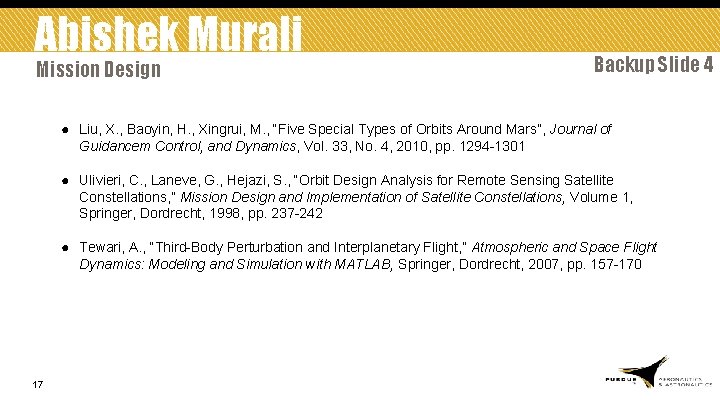
Abishek Murali Mission Design Backup Slide 4 ● Liu, X. , Baoyin, H. , Xingrui, M. , “Five Special Types of Orbits Around Mars”, Journal of Guidancem Control, and Dynamics, Vol. 33, No. 4, 2010, pp. 1294 -1301 ● Ulivieri, C. , Laneve, G. , Hejazi, S. , “Orbit Design Analysis for Remote Sensing Satellite Constellations, ” Mission Design and Implementation of Satellite Constellations, Volume 1, Springer, Dordrecht, 1998, pp. 237 -242 ● Tewari, A. , “Third-Body Perturbation and Interplanetary Flight, ” Atmospheric and Space Flight Dynamics: Modeling and Simulation with MATLAB, Springer, Dordrecht, 2007, pp. 157 -170 17
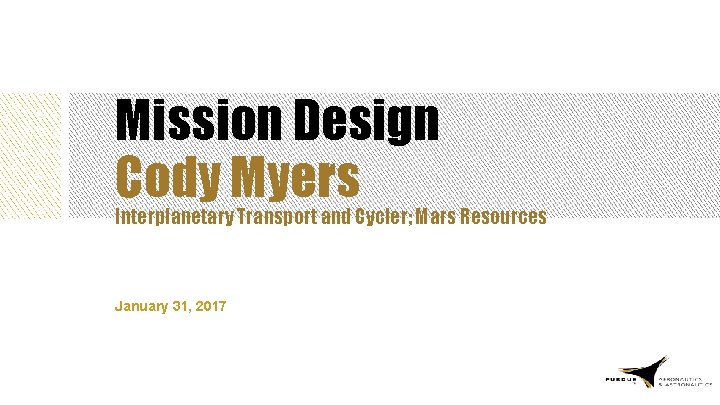
Mission Design Cody Myers Interplanetary Transport and Cycler; Mars Resources January 31, 2017
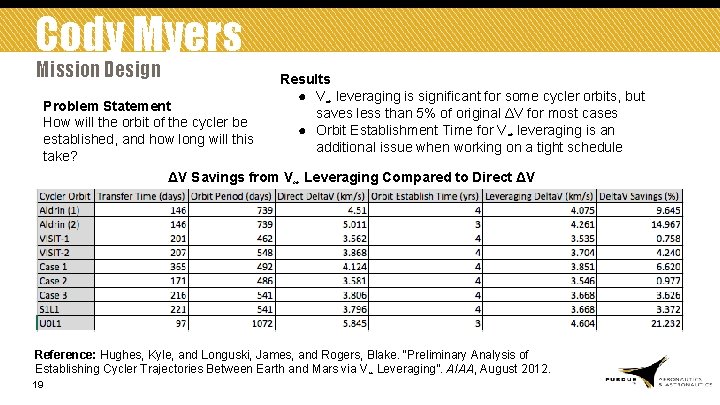
Cody Myers Mission Design Problem Statement How will the orbit of the cycler be established, and how long will this take? Results ● V∞ leveraging is significant for some cycler orbits, but saves less than 5% of original ΔV for most cases ● Orbit Establishment Time for V∞ leveraging is an additional issue when working on a tight schedule ΔV Savings from V∞ Leveraging Compared to Direct ΔV Reference: Hughes, Kyle, and Longuski, James, and Rogers, Blake. “Preliminary Analysis of Establishing Cycler Trajectories Between Earth and Mars via V∞ Leveraging”. AIAA, August 2012. 19
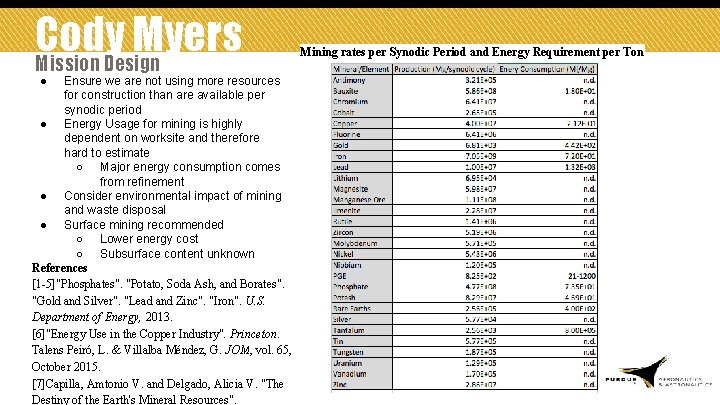
Cody Myers Mission Design ● Ensure we are not using more resources for construction than are available per synodic period ● Energy Usage for mining is highly dependent on worksite and therefore hard to estimate ○ Major energy consumption comes from refinement ● Consider environmental impact of mining and waste disposal ● Surface mining recommended ○ Lower energy cost ○ Subsurface content unknown References [1 -5]"Phosphates". "Potato, Soda Ash, and Borates". "Gold and Silver". "Lead and Zinc". "Iron". U. S. Department of Energy, 2013. [6]"Energy Use in the Copper Industry". Princeton. Talens Peiró, L. & Villalba Méndez, G. JOM, vol. 65, October 2015. [7]Capilla, Amtonio V. and Delgado, Alicia V. "The 20 Destiny of the Earth's Mineral Resources". Mining rates per Synodic Period and Energy Requirement per Ton
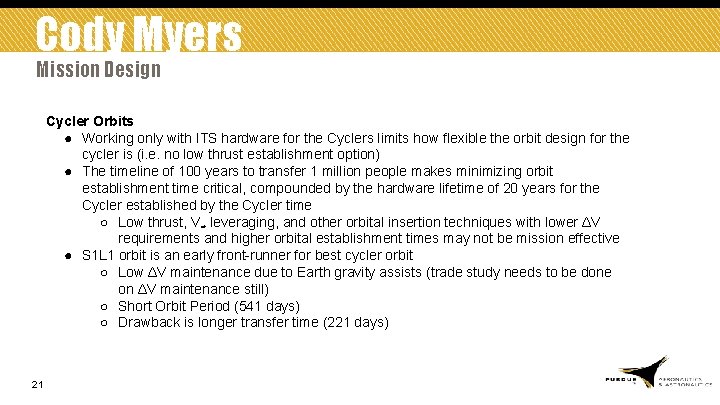
Cody Myers Mission Design Cycler Orbits ● Working only with ITS hardware for the Cyclers limits how flexible the orbit design for the cycler is (i. e. no low thrust establishment option) ● The timeline of 100 years to transfer 1 million people makes minimizing orbit establishment time critical, compounded by the hardware lifetime of 20 years for the Cycler established by the Cycler time ○ Low thrust, V∞ leveraging, and other orbital insertion techniques with lower ΔV requirements and higher orbital establishment times may not be mission effective ● S 1 L 1 orbit is an early front-runner for best cycler orbit ○ Low ΔV maintenance due to Earth gravity assists (trade study needs to be done on ΔV maintenance still) ○ Short Orbit Period (541 days) ○ Drawback is longer transfer time (221 days) 21
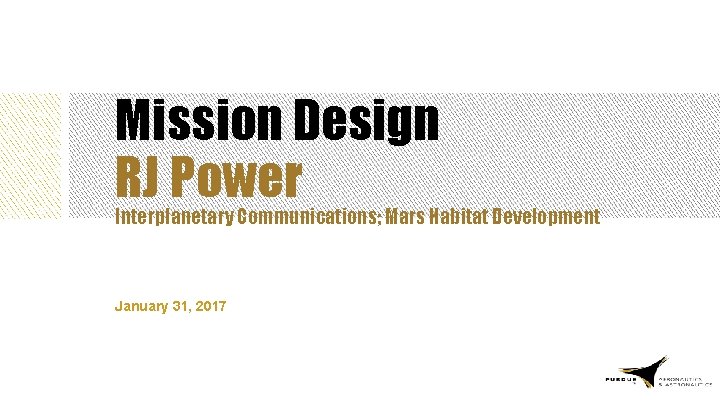
Mission Design RJ Power Interplanetary Communications; Mars Habitat Development January 31, 2017
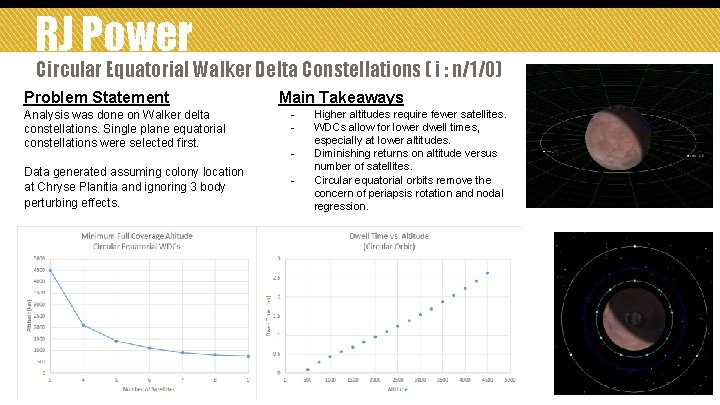
RJ Power Circular Equatorial Walker Delta Constellations ( i : n/1/0) Problem Statement Analysis was done on Walker delta constellations. Single plane equatorial constellations were selected first. Data generated assuming colony location at Chryse Planitia and ignoring 3 body perturbing effects. 23 Main Takeaways - Higher altitudes require fewer satellites. WDCs allow for lower dwell times, especially at lower altitudes. Diminishing returns on altitude versus number of satellites. Circular equatorial orbits remove the concern of periapsis rotation and nodal regression.
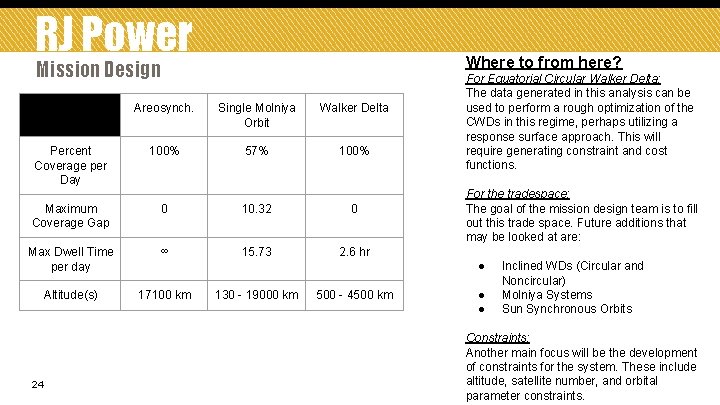
RJ Power Where to from here? Mission Design Percent Coverage per Day Areosynch. Single Molniya Orbit Walker Delta 100% 57% 100% Maximum Coverage Gap 0 10. 32 0 Max Dwell Time per day ∞ 15. 73 2. 6 hr Altitude(s) 17100 km 24 For Equatorial Circular Walker Delta: The data generated in this analysis can be used to perform a rough optimization of the CWDs in this regime, perhaps utilizing a response surface approach. This will require generating constraint and cost functions. For the tradespace: The goal of the mission design team is to fill out this trade space. Future additions that may be looked at are: ● 130 - 19000 km 500 - 4500 km ● ● Inclined WDs (Circular and Noncircular) Molniya Systems Sun Synchronous Orbits Constraints: Another main focus will be the development of constraints for the system. These include altitude, satellite number, and orbital parameter constraints.
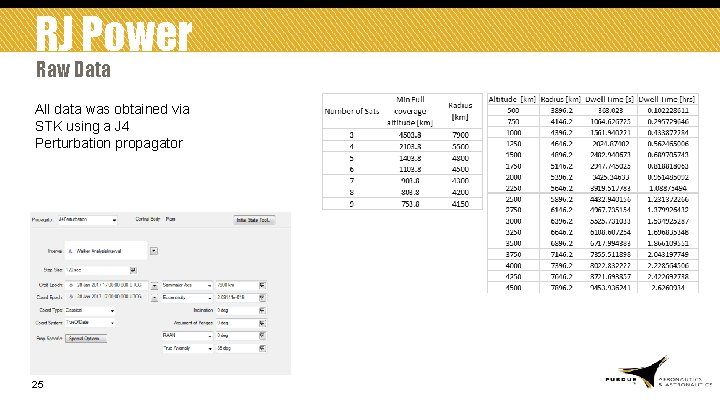
RJ Power Raw Data All data was obtained via STK using a J 4 Perturbation propagator 25
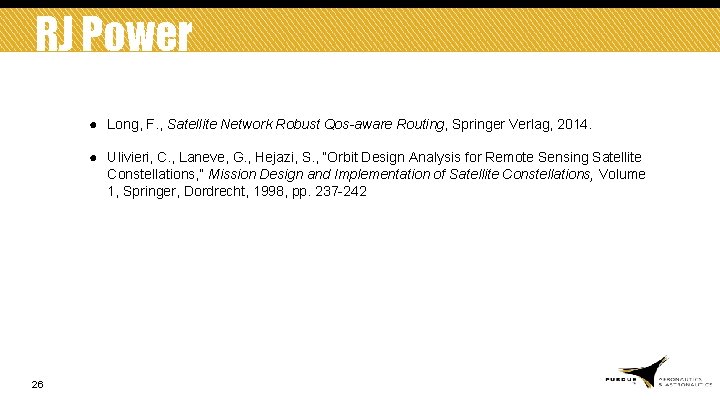
RJ Power ● Long, F. , Satellite Network Robust Qos-aware Routing, Springer Verlag, 2014. ● Ulivieri, C. , Laneve, G. , Hejazi, S. , “Orbit Design Analysis for Remote Sensing Satellite Constellations, ” Mission Design and Implementation of Satellite Constellations, Volume 1, Springer, Dordrecht, 1998, pp. 237 -242 26
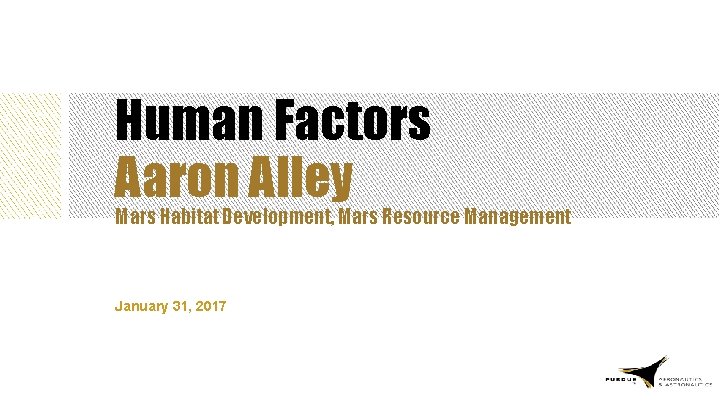
Human Factors Aaron Alley Mars Habitat Development, Mars Resource Management January 31, 2017
![Aaron Alley Human Factors Dorms 9 6 m2 1 5 Purdue Aaron Alley Human Factors ● Dorms ○ 9. 6 m^2 [1 -5] ● Purdue](https://slidetodoc.com/presentation_image_h/184fd3da0ebff5691a469b53cfd00971/image-28.jpg)
Aaron Alley Human Factors ● Dorms ○ 9. 6 m^2 [1 -5] ● Purdue dorm ○ Hawkins Hall (single room) ■ 11. 6 m^2 ○ Cary (single room) ■ 11. 8 m^2 ○ First Street (single room) ■ 15 m^2 [6] 28 Variable name Value Units Personal living area 10 m^2 / colonist Total personal living area 1000 m^2
![Aaron Alley Human Factors Hostel 7 6 5 m2 NYC single Aaron Alley Human Factors ● Hostel [7] ○ 6. 5 m^2 ● NYC single](https://slidetodoc.com/presentation_image_h/184fd3da0ebff5691a469b53cfd00971/image-29.jpg)
Aaron Alley Human Factors ● Hostel [7] ○ 6. 5 m^2 ● NYC single housing ○ 7. 4 m^2 ● UK single housing ○ 6. 5 m^2 [8] ● American vs. Japanese housing area ○ Average American single room ■ 9. 3 - 10. 6 m^2 [9] ○ Average Japanese single room ■ 9. 9 m^2 [10] 29
![Aaron Alley Human Factors 1 Room Layouts Concordia University online http www cune Aaron Alley Human Factors [1] "Room Layouts, " Concordia University [online]. http: //www. cune.](https://slidetodoc.com/presentation_image_h/184fd3da0ebff5691a469b53cfd00971/image-30.jpg)
Aaron Alley Human Factors [1] "Room Layouts, " Concordia University [online]. http: //www. cune. edu/student-life/residence-hall-rooms/ [retrieved 24 January 2017]. [2] "SD Mines, " South Dakota School of Mines & Technology [online]. http: //www. sdsmt. edu/Campus-Life/Housing-and. Dining/Residence-Life/Housing-Information/Room-Dimensions/ [retrieved 24 January 2017]. [3] "University Housing, " Southern Illinois University [online]. https: //www. siue. edu/housing/woodland/room-dimensions. shtml [retrieved 24 January 2017]. [4] "Housing and Resident Life, " University of Southern Indiana [online]. https: //www. usi. edu/housing/frequently-asked-questions/moving -to-campus/room-size/ [retrieved 24 January 2017]. [5] "University Residences, " Purdue University [online]. https: //www. housing. purdue. edu/Housing/Residences/Earhart/layout. html [retrieved 24 January 2017]. [6] "University Residences, " Purdue University [online]. https: //www. housing. purdue. edu/Housing/Residences/index. html [retrieved 24 January 2017]. [7] architecture CAD “Room Sizes” [Online database] URL: http: //www 2. d 125. org/im/ACAD/pdf/room_sizes. pdf[cited 24 January 2017] [8] The Royal Borough of Kensington and Chelsea “Houses in multiple occupation”[online]. https: //www. rbkc. gov. uk/pdf/HMO%20 standards%20 hostels%20 and%20 staff%20 accommodation. pdf [retrieved 24 January 2017] [9] New York city government “Chapter 2 housing maintenance code” New York city RGB [online]. http: //www. nycrgb. org/html/resources/hmc/sub 3/art 4. html [retrieved 24 January 2017]. [10] All about teaching English in Japan. com “Take a peek inside a “Typical” Tokyo Apatment” [online]. http: //www. all-about-teachingenglish-in-japan. com/Tokyoapartment. html[retrieved 24 January 2017] 30
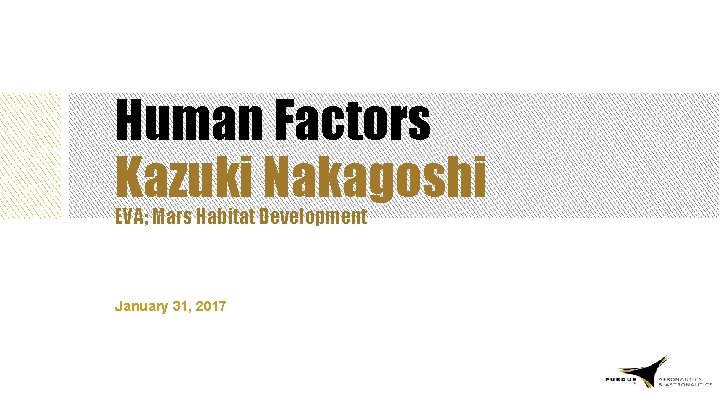
Human Factors Kazuki Nakagoshi EVA; Mars Habitat Development January 31, 2017
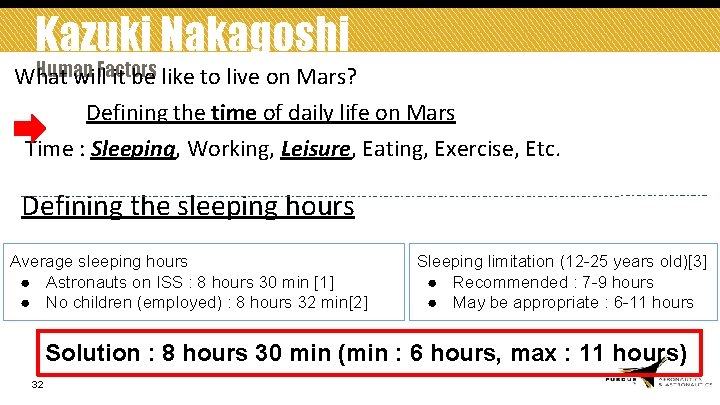
Kazuki Nakagoshi Human What will. Factors it be like to live on Mars? Defining the time of daily life on Mars Time : Sleeping, Working, Leisure, Eating, Exercise, Etc. Defining the sleeping hours Kazuki Nakagoshi Average sleeping hours ● Astronauts on ISS : 8 hours 30 min [1] ● No children (employed) : 8 hours 32 min[2] Sleeping limitation (12 -25 years old)[3] ● Recommended : 7 -9 hours ● May be appropriate : 6 -11 hours Solution : 8 hours 30 min (min : 6 hours, max : 11 hours) 32
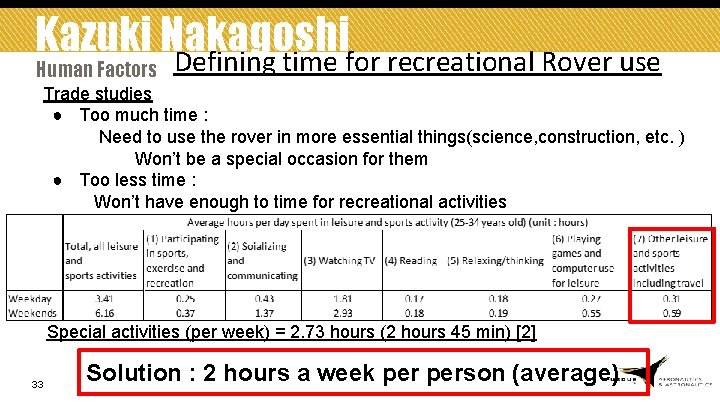
Kazuki Nakagoshi Defining time for recreational Rover use Human Factors Trade studies ● Too much time : Need to use the rover in more essential things(science, construction, etc. ) Won’t be a special occasion for them ● Too less time : Won’t have enough to time for recreational activities Special activities (per week) = 2. 73 hours (2 hours 45 min) [2] 33 Solution : 2 hours a week person (average)
![Kazuki Nakagoshi Human Factors Comparison of daily life between astronaut and nonhousehold employer 1 Kazuki Nakagoshi Human Factors Comparison of daily life between astronaut and nonhousehold employer [1],](https://slidetodoc.com/presentation_image_h/184fd3da0ebff5691a469b53cfd00971/image-34.jpg)
Kazuki Nakagoshi Human Factors Comparison of daily life between astronaut and nonhousehold employer [1], [2] 34
![Kazuki Nakagoshi Human Factors Reference 1NASA International Space Station Timelines Jan 16 2014 online Kazuki Nakagoshi Human Factors Reference [1]NASA, “International Space Station Timelines” Jan. 16, 2014 [online](https://slidetodoc.com/presentation_image_h/184fd3da0ebff5691a469b53cfd00971/image-35.jpg)
Kazuki Nakagoshi Human Factors Reference [1]NASA, “International Space Station Timelines” Jan. 16, 2014 [online database] https: //www. nasa. gov/sites/default/files/011614_tl. pdf [cited 30 January 2017] [2] U. S. Department of labor, “American Time Use Survey— 2015 Results” Bureau of labor statistics [online database] https: //www. bls. gov/news. release/pdf/atus. pdf [cited 24 January 2017] 35 [3]Max Hirshkowitz, Ph. D, Kaitlyn Whiton, MHS, Steven M. Albert, Ph. D, Cathy Alessi, MD, Oliviero Bruni, MD, Lydia Don. Carlos, Ph. D, Nancy Hazen, Ph. D, John Herman, Ph. D, Eliot S. Katz, MD, Leila Kheirandish-Gozal, MD, MSc, David N. Neubauer, MD, Anne E. O’Donnell, MD, Maurice Ohayon, MD, DSc, Ph. D, John Peever, Ph. D, Robert Rawding, Ph. D, Ramesh C. Sachdeva, MD, Ph. D, JD, Belinda Setters, MD, Michael V. Vitiello, Ph. D, J. Catesby Ware, Ph. D, Paula J. Adams Hillard, MD, “Sleep health-National Sleep Foundation’s sleep time duration recommendations: methodology and results summary” Journal of the National Sleep Foundation. Published by Elsevier Inc.
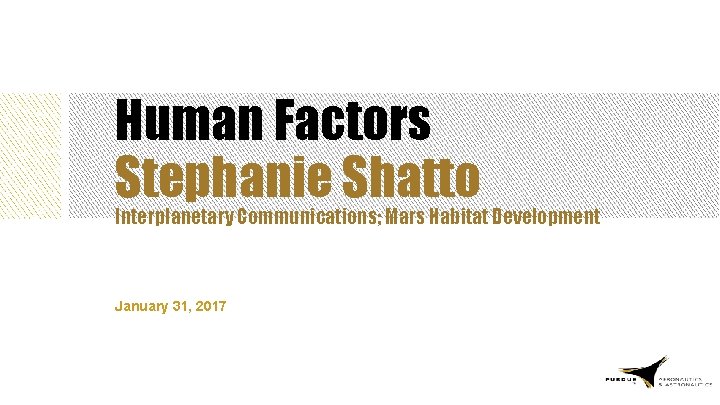
Human Factors Stephanie Shatto Interplanetary Communications; Mars Habitat Development January 31, 2017
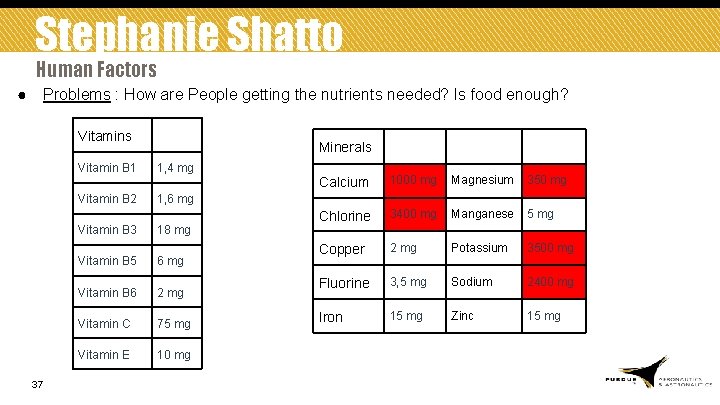
Stephanie Shatto Human Factors ● Problems : How are People getting the nutrients needed? Is food enough? Vitamins 37 Minerals Vitamin B 1 1, 4 mg Vitamin B 2 1, 6 mg Vitamin B 3 18 mg Vitamin B 5 6 mg Vitamin B 6 2 mg Vitamin C 75 mg Vitamin E 10 mg Calcium 1000 mg Magnesium 350 mg Chlorine 3400 mg Manganese 5 mg Copper 2 mg Potassium 3500 mg Fluorine 3, 5 mg Sodium 2400 mg Iron 15 mg Zinc 15 mg
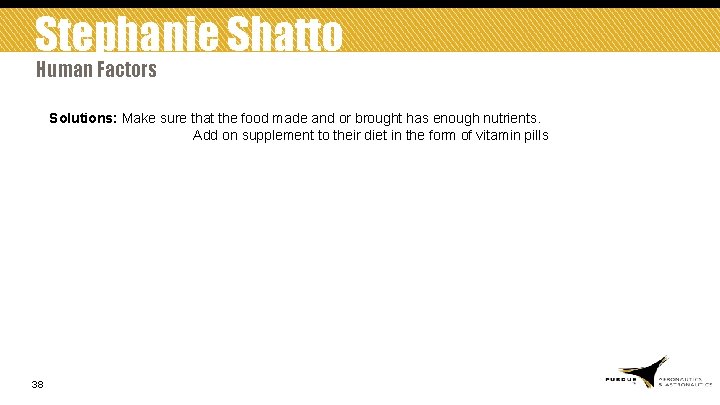
Stephanie Shatto Human Factors Solutions: Make sure that the food made and or brought has enough nutrients. Add on supplement to their diet in the form of vitamin pills 38
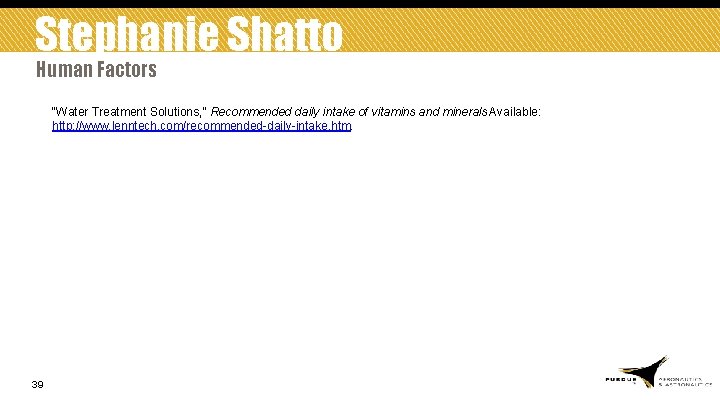
Stephanie Shatto Human Factors “Water Treatment Solutions, ” Recommended daily intake of vitamins and minerals Available: http: //www. lenntech. com/recommended-daily-intake. htm. 39
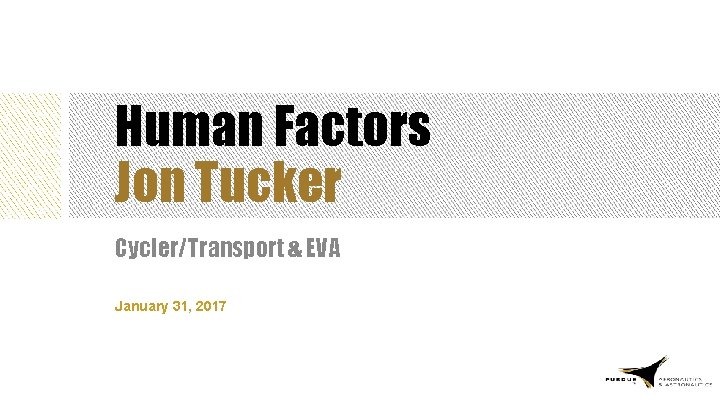
Human Factors Jon Tucker Cycler/Transport & EVA January 31, 2017
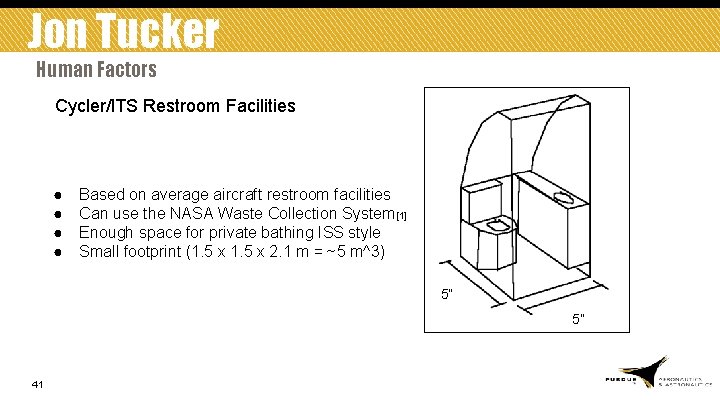
Jon Tucker Human Factors Cycler/ITS Restroom Facilities ● ● Based on average aircraft restroom facilities Can use the NASA Waste Collection System[1] Enough space for private bathing ISS style Small footprint (1. 5 x 2. 1 m = ~5 m^3) 5” 5” 41
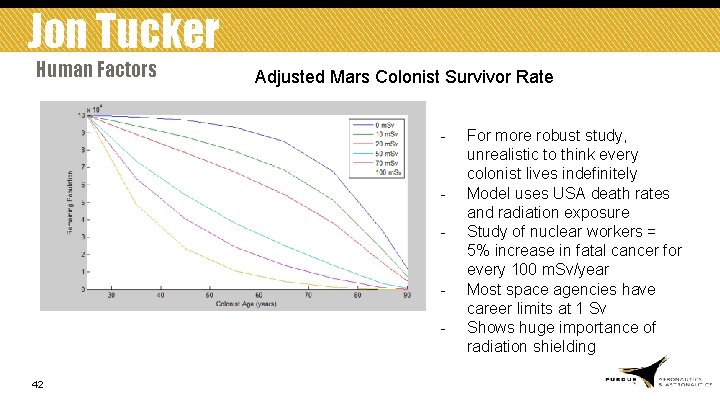
Jon Tucker Human Factors Adjusted Mars Colonist Survivor Rate - 42 For more robust study, unrealistic to think every colonist lives indefinitely Model uses USA death rates and radiation exposure Study of nuclear workers = 5% increase in fatal cancer for every 100 m. Sv/year Most space agencies have career limits at 1 Sv Shows huge importance of radiation shielding
![Jon Tucker Human Factors References 1 Waste Collection System https spaceflight nasa govshuttlereferenceshutreforbitereclsswcs Jon Tucker Human Factors References [1] “Waste Collection System, ” https: //spaceflight. nasa. gov/shuttle/reference//shutref/orbiter/eclss/wcs.](https://slidetodoc.com/presentation_image_h/184fd3da0ebff5691a469b53cfd00971/image-43.jpg)
Jon Tucker Human Factors References [1] “Waste Collection System, ” https: //spaceflight. nasa. gov/shuttle/reference//shutref/orbiter/eclss/wcs. html [retrieved 26 Jan. 2017] [2] “Death rates by 10 -year age groups, ” National Vital Statistics System [online], No. 20, 2010, https: //www. cdc. gov/nchs/data/dvs/mortfinal 2007_worktable 23 r. pdf [retrieved Jan. 24, 2017]. [3] "Radiation and Life, " World Nuclear Association [online]. http: //www. worldnuclear. org/information-library/safety-and-security/radiation-and-health/radiation-and-life. aspx [retrieved 23 January 2017]. 43
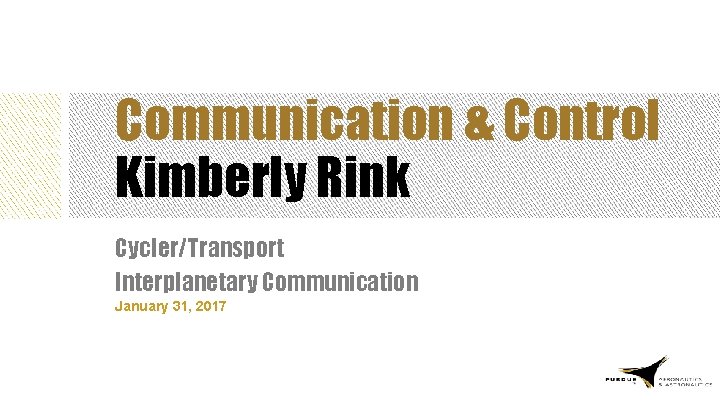
Communication & Control Kimberly Rink Cycler/Transport Interplanetary Communication January 31, 2017
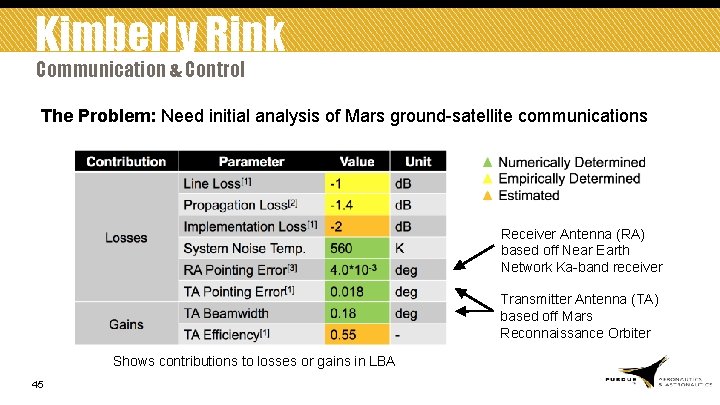
Kimberly Rink Communication & Control The Problem: Need initial analysis of Mars ground-satellite communications Receiver Antenna (RA) based off Near Earth Network Ka-band receiver Transmitter Antenna (TA) based off Mars Reconnaissance Orbiter Shows contributions to losses or gains in LBA 45
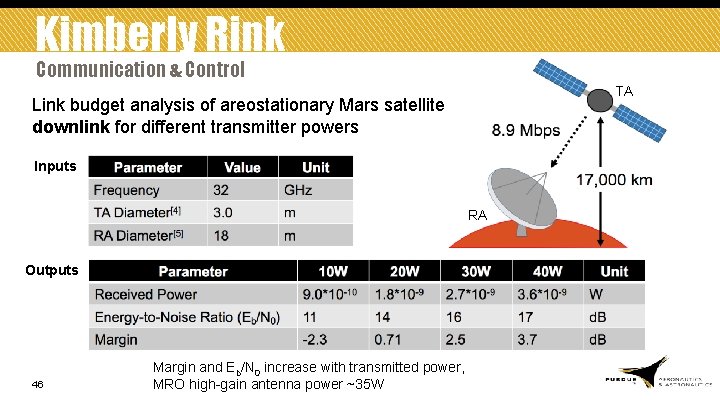
Kimberly Rink Communication & Control TA Link budget analysis of areostationary Mars satellite downlink for different transmitter powers Inputs RA Outputs 46 Margin and Eb/N 0 increase with transmitted power, MRO high-gain antenna power ~35 W
![Kimberly Rink Communication Control Backup Slide References 1 Larson W and Wertz J Kimberly Rink Communication & Control Backup Slide References: [1] Larson, W. and Wertz, J.](https://slidetodoc.com/presentation_image_h/184fd3da0ebff5691a469b53cfd00971/image-47.jpg)
Kimberly Rink Communication & Control Backup Slide References: [1] Larson, W. and Wertz, J. , “Communications Architecture, ” Space Analysis and Design, 3 rd ed. , Microcosm Press, El Segundo, CA, 1999. [2] Hemmati, H. , “Propagation Issues for Communication Between Earth and Mars”, Jet Propulsion Laboratory DESCANSO, 2005. [3] “Antenna Positioning”, Deep Space Network, Jet Propulsion Laboratory, Rev. C, 09 February, 2015. [4] “Mars Reconnaissance Orbiter Fact Sheet”, NASA Jet Propulsion Laboratory. [5] “Near Earth Network (NEN) User’s Guide”, Rev. 2, 24 February, 2016. 47
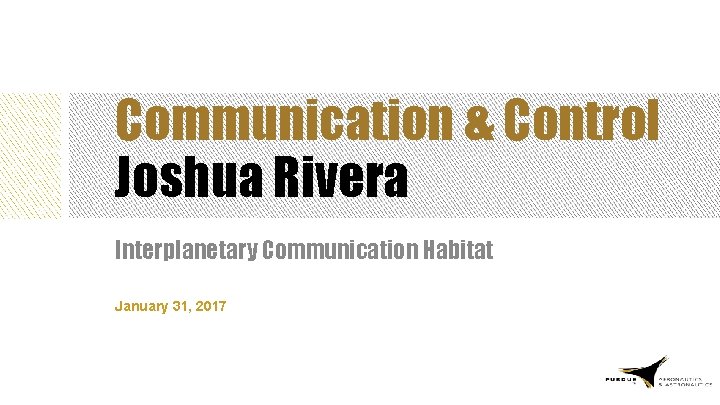
Communication & Control Joshua Rivera Interplanetary Communication Habitat January 31, 2017
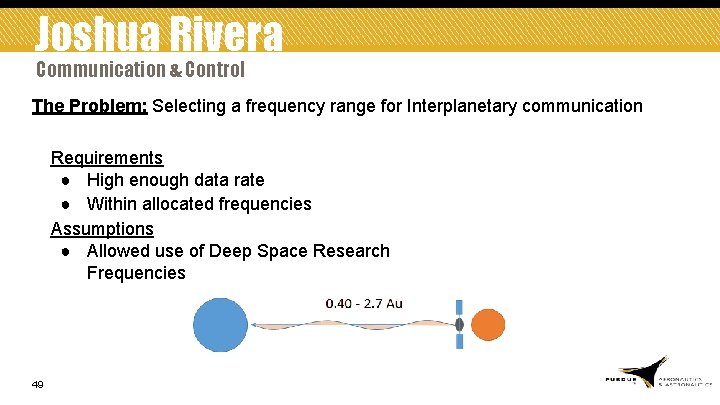
Joshua Rivera Communication & Control The Problem: Selecting a frequency range for Interplanetary communication Requirements ● High enough data rate ● Within allocated frequencies Assumptions ● Allowed use of Deep Space Research Frequencies 49
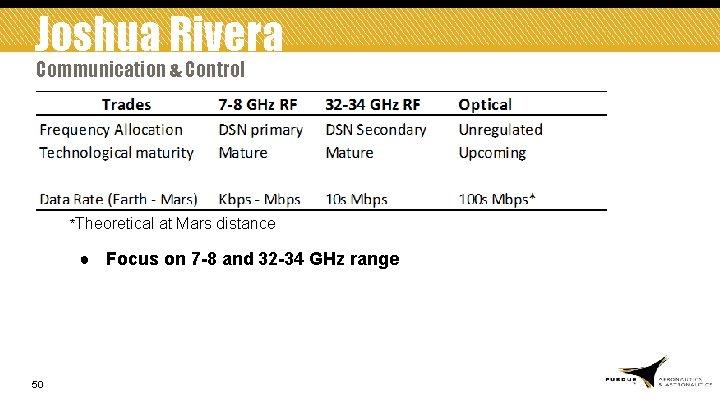
Joshua Rivera Communication & Control *Theoretical at Mars distance ● Focus on 7 -8 and 32 -34 GHz range 50
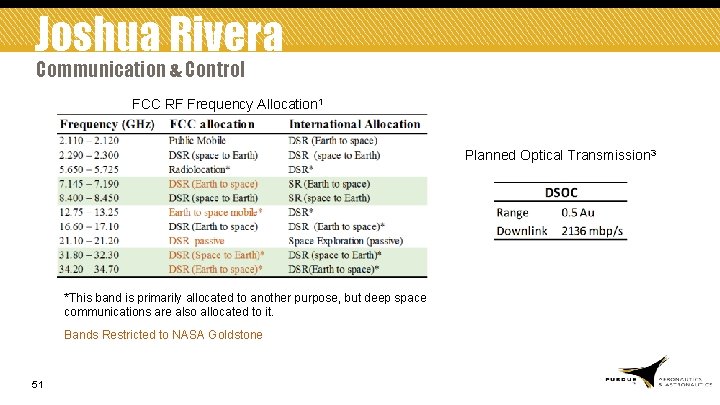
Joshua Rivera Communication & Control FCC RF Frequency Allocation 1 Planned Optical Transmission 3 *This band is primarily allocated to another purpose, but deep space communications are also allocated to it. Bands Restricted to NASA Goldstone 51
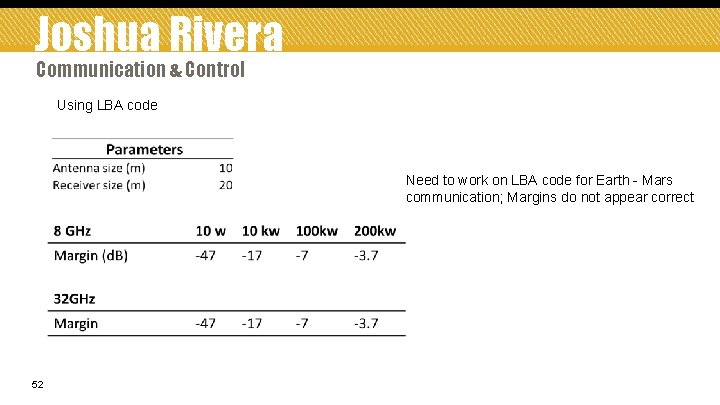
Joshua Rivera Communication & Control Using LBA code Need to work on LBA code for Earth - Mars communication; Margins do not appear correct 52
![Joshua Rivera Communication Control References 1 FCC Online Table of Frequency Allocations Joshua Rivera Communication & Control References [1] “FCC Online Table of Frequency Allocations, ”](https://slidetodoc.com/presentation_image_h/184fd3da0ebff5691a469b53cfd00971/image-53.jpg)
Joshua Rivera Communication & Control References [1] “FCC Online Table of Frequency Allocations, ” Federal Communications Commission Office of Engineering and Technology Policy and Rules Division. ” 47 C. F. R. § 2. 106, Revised Aug. 2016. URL: https: //transition. fcc. gov/oet/spectrum/table/fcctable. pdf [cited January 2017]. [2] Mai, T. , ed. , “Benefits of Optical Communications, ” NASA. gov URL: https: //www. nasa. gov/directorates/heo/scan/engineering/technology/txt_opticalcomm_benefits. html [cited January 2017]. [3]“Game Changing Development Deep Space Optical Communications (DSOC), ” NASA Jet Propulsion Laboratory, Pasadena, CA. Aug. 2015. URL: https: //www. nasa. gov/sites/default/files/atoms/files/fs_dsoc_factsheet_150910. pdf [cited January 2017] [4] Larson, W. and Wertz, J. , “Communications Architecture, ” Space Analysis and Design, 3 rd ed. , Microcosm Press, El Segundo, CA, 1999. 53
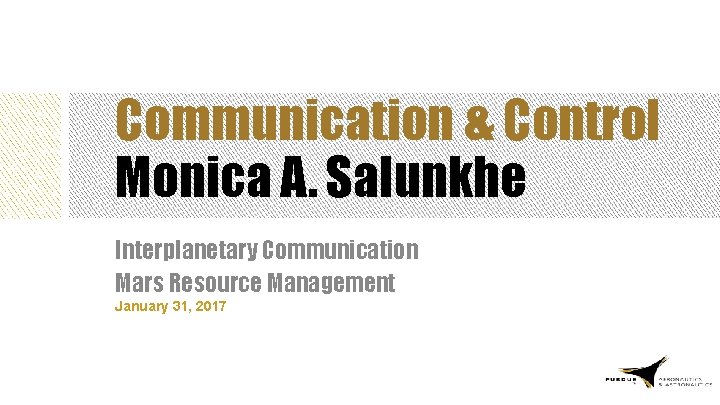
Communication & Control Monica A. Salunkhe Interplanetary Communication Mars Resource Management January 31, 2017
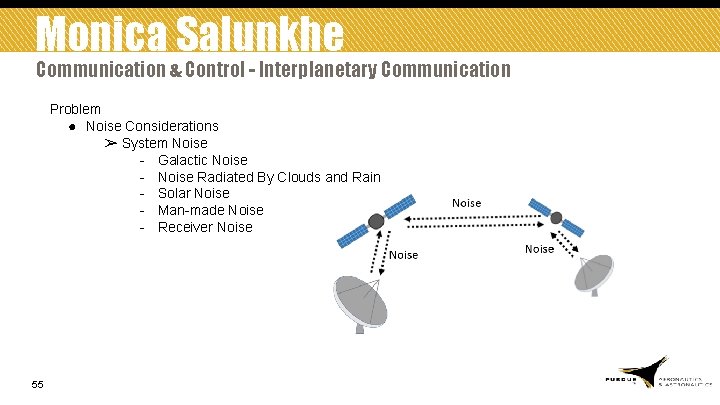
Monica Salunkhe Communication & Control - Interplanetary Communication Problem ● Noise Considerations ➢ System Noise - Galactic Noise - Noise Radiated By Clouds and Rain - Solar Noise - Man-made Noise - Receiver Noise 55
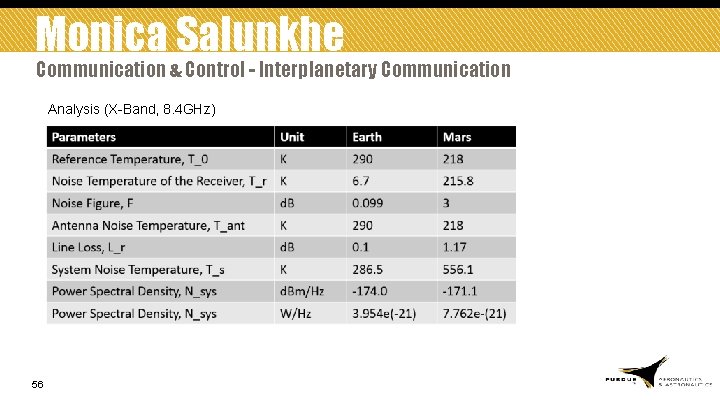
Monica Salunkhe Communication & Control - Interplanetary Communication Analysis (X-Band, 8. 4 GHz) 56
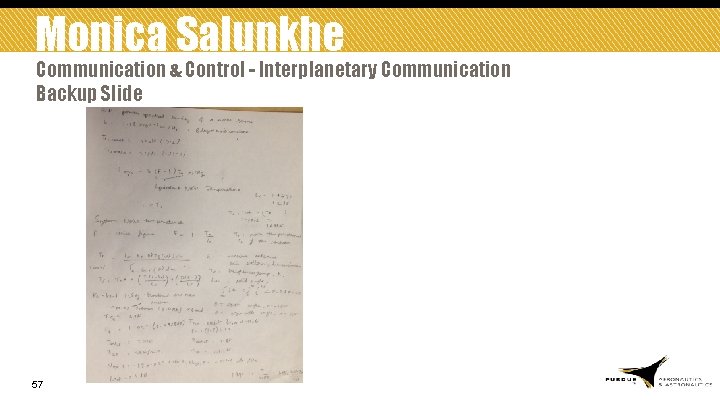
Monica Salunkhe Communication & Control - Interplanetary Communication Backup Slide 57
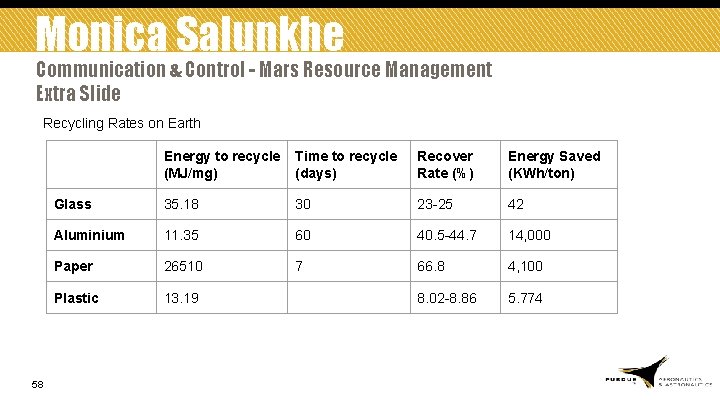
Monica Salunkhe Communication & Control - Mars Resource Management Extra Slide Recycling Rates on Earth 58 Energy to recycle (MJ/mg) Time to recycle (days) Recover Rate (%) Energy Saved (KWh/ton) Glass 35. 18 30 23 -25 42 Aluminium 11. 35 60 40. 5 -44. 7 14, 000 Paper 26510 7 66. 8 4, 100 Plastic 13. 19 8. 02 -8. 86 5. 774
![Monica Salunkhe Communication Control References 1 Larson W and Wertz J Communications Monica Salunkhe Communication & Control References [1] Larson, W. and Wertz, J. , “Communications](https://slidetodoc.com/presentation_image_h/184fd3da0ebff5691a469b53cfd00971/image-59.jpg)
Monica Salunkhe Communication & Control References [1] Larson, W. and Wertz, J. , “Communications Architecture, ” Space Analysis and Design, 3 rd ed. , Microcosm Press, El Segundo, CA, 1999. [2] Tsai, M. , “Link Budget”, Nanyang Technological University, 22 Sept. 2011. [3] Sue, M. K. , Hansen, D. , Ho, C. , and Peng, T. , “Frequencies for Mars Local Communications”, NASA, 2014. [4] Ho, C. , Slobin, S. , Sue, M. , and Njoku, E. , “Mars Background Noise Temperatures Received by Spacecraft Antennas”, NASA, 15 May 2002. [5] Otoshi, T. Y. , “Calculation of Antenna System Noise Temperatures at Different Ports-Revisited”, NASA, 15 Aug. 2002. [6] Stelzried, C. , Freiley, A. J. , and Reid, M. S. , “System Noise Concepts with DSN Applications”, NASA, [retrieved 30 Jan. 2017]. [7] “Buildings & Grounds Maintenance, ” Frequently Asked Questions: Benefits of Recycling | Buildings & Grounds Maintenance Available: http: //bgm. stanford. edu/pssi_faq_benefits. 59
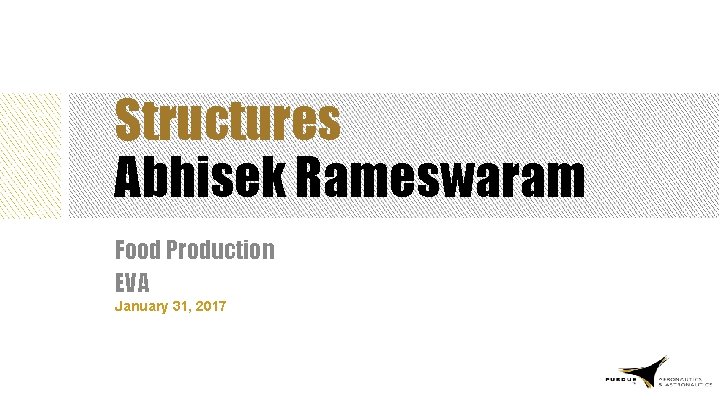
Structures Abhisek Rameswaram Food Production EVA January 31, 2017
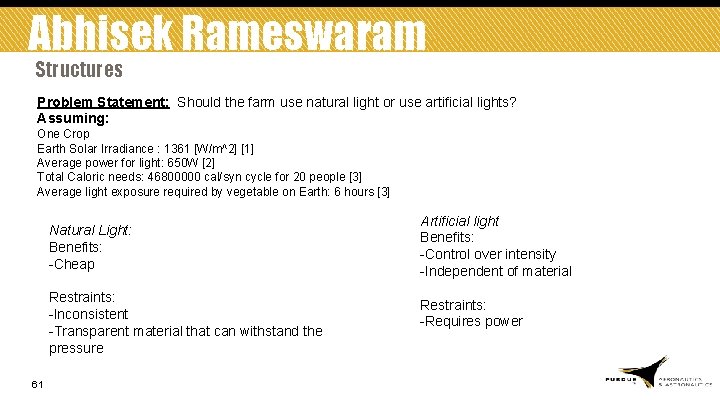
Abhisek Rameswaram Structures Problem Statement: Should the farm use natural light or use artificial lights? Assuming: One Crop Earth Solar Irradiance : 1361 [W/m^2] [1] Average power for light: 650 W [2] Total Caloric needs: 46800000 cal/syn cycle for 20 people [3] Average light exposure required by vegetable on Earth: 6 hours [3] Natural Light: Benefits: -Cheap Restraints: -Inconsistent -Transparent material that can withstand the pressure 61 Artificial light Benefits: -Control over intensity -Independent of material Restraints: -Requires power
![Abhisek Rameswaram Structures Table 1 Size Requirements 3 Food Calm2 Farm Size m2 Potatos Abhisek Rameswaram Structures Table 1: Size Requirements [3] Food Cal/m^2 Farm Size [m^2] Potatos](https://slidetodoc.com/presentation_image_h/184fd3da0ebff5691a469b53cfd00971/image-62.jpg)
Abhisek Rameswaram Structures Table 1: Size Requirements [3] Food Cal/m^2 Farm Size [m^2] Potatos 5361. 62 8728. 71 Carrots 3875. 01 12077. 39 Onions 1452. 6 32218. 09 Energy/m^2 [k. Wh/m^2] Total Energy Required [k. Wh] Number of Lights 8. 17 71278. 61 18277 8. 17 98623. 95 25288 8. 17 263092. 94 67460 Table 2: Energy Requirements Volume [m^3] Mass [kg] 545. 09 298443. 5237 754. 21 412938. 4868 2011. 95 1101570. 126 Table 3: K 5 Series XL 1000 Indoor LED Grow [2] 62 Conclusion: Using transparent materials and natural lighting Going Forward: -Glass -Polycarbonate (PC) -Fiber Glass
![Abhisek Rameswaram Structures Back Up References 1NASA Mars Fact Sheet Available http Abhisek Rameswaram Structures Back. Up References: [1]NASA. , “Mars Fact Sheet, ” Available: http:](https://slidetodoc.com/presentation_image_h/184fd3da0ebff5691a469b53cfd00971/image-63.jpg)
Abhisek Rameswaram Structures Back. Up References: [1]NASA. , “Mars Fact Sheet, ” Available: http: //nssdc. gsfc. nasa. gov/ planetary/factsheet/marsfact. html [2] “K 5 Series XL 1000 Indoor LED Grow Lights, ” Kind Led Grow Lights Available: https: //www. kindledgrowlights. com/p roducts/k 5 -series-xl 1000 -indoor-ledgrow-lights-best-led-grow-lights. [3] “Short Report 1: 100 Person Base Requirements, ” 2017 63
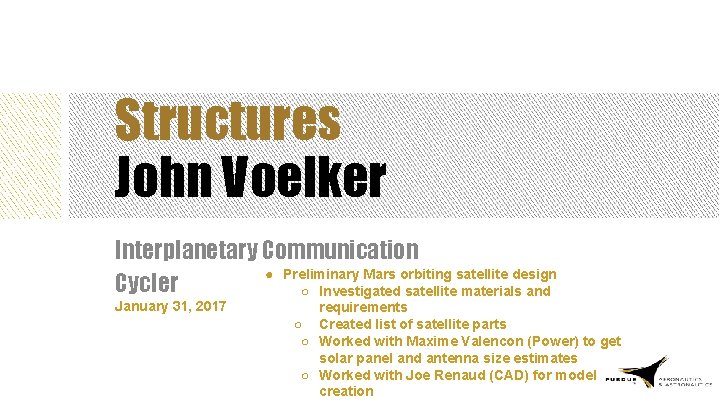
Structures John Voelker Interplanetary Communication ● Preliminary Mars orbiting satellite design Cycler ○ Investigated satellite materials and January 31, 2017 requirements ○ Created list of satellite parts ○ Worked with Maxime Valencon (Power) to get solar panel and antenna size estimates ○ Worked with Joe Renaud (CAD) for model creation
![John Voelker Structures Interplanetary Communication Material MassArea gcm2 Layup Position Beta Cloth 0 John Voelker Structures & Interplanetary Communication Material Mass/Area [g/cm^2] Layup Position Beta Cloth 0.](https://slidetodoc.com/presentation_image_h/184fd3da0ebff5691a469b53cfd00971/image-65.jpg)
John Voelker Structures & Interplanetary Communication Material Mass/Area [g/cm^2] Layup Position Beta Cloth 0. 0237 Outer Cover Kapton 0. 0019 -0. 019 Outer Cover, Reflector, Inner cover Teflon 0. 0028 -0. 055 Outer Cover, Reflector Mylar 0. 0007 -0. 0175 Reflector Polyester - Polyester Dacron 5. 45 -7. 15 Spacer Nomex 5. 45 -7. 15 Spacer Material Layup Outer Cover Reflector Repeated Spacer 15 -20 layers Reflector Inner Cover 65 [1] & [2]
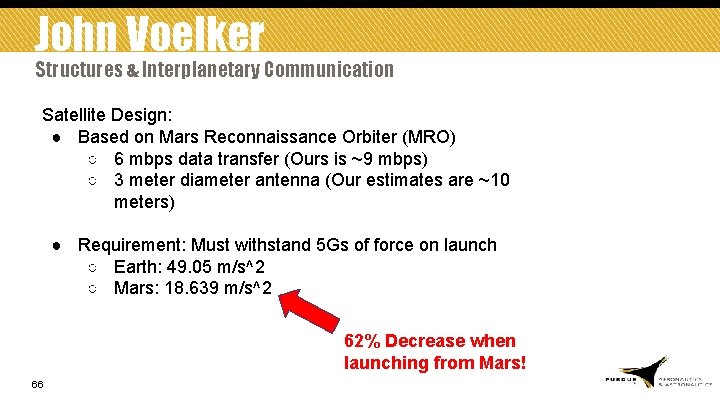
John Voelker Structures & Interplanetary Communication Satellite Design: ● Based on Mars Reconnaissance Orbiter (MRO) ○ 6 mbps data transfer (Ours is ~9 mbps) ○ 3 meter diameter antenna (Our estimates are ~10 meters) ● Requirement: Must withstand 5 Gs of force on launch ○ Earth: 49. 05 m/s^2 ○ Mars: 18. 639 m/s^2 62% Decrease when launching from Mars! 66
![John Voelker Structures Interplanetary Communication References 1 Dooling D and Finckenor M John Voelker Structures & Interplanetary Communication References [1] Dooling, D. , and Finckenor, M.](https://slidetodoc.com/presentation_image_h/184fd3da0ebff5691a469b53cfd00971/image-67.jpg)
John Voelker Structures & Interplanetary Communication References [1] Dooling, D. , and Finckenor, M. M. , “Multilayer Insulation Material Guidelines, ” NASA TP-209263, 1999. [2] Fisher, D. , “Typical Onboard Systems, ” NASA JPL: Basics of Space Flight Available: http: //solarsystem. nasa. gov/basics/bsf 11 -4. php. 67
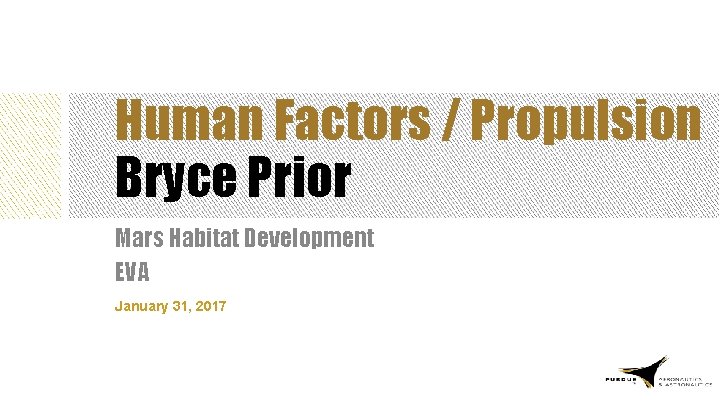
Human Factors / Propulsion Bryce Prior Mars Habitat Development EVA January 31, 2017
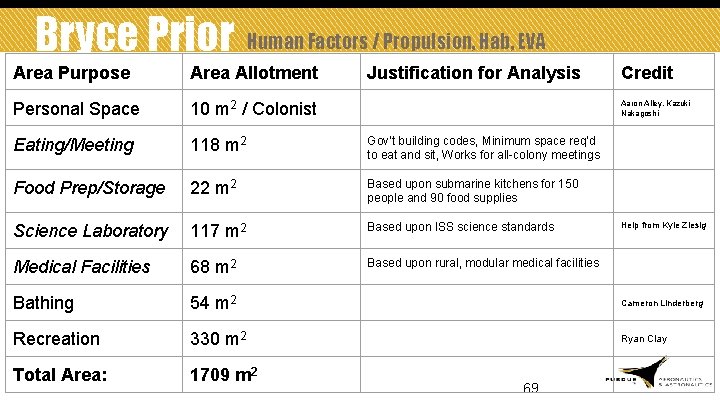
Bryce Prior Human Factors / Propulsion, Hab, EVA Area Purpose Area Allotment Justification for Analysis Credit Personal Space 10 m 2 / Colonist Eating/Meeting 118 m 2 Gov’t building codes, Minimum space req’d to eat and sit, Works for all-colony meetings Food Prep/Storage 22 m 2 Based upon submarine kitchens for 150 people and 90 food supplies Science Laboratory 117 m 2 Based upon ISS science standards Medical Facilities 68 m 2 Based upon rural, modular medical facilities Bathing 54 m 2 Cameron Linderberg Recreation 330 m 2 Ryan Clay Total Area: 1709 m 2 Aaron Alley, Kazuki Nakagoshi 69 Help from Kyle Ziesig
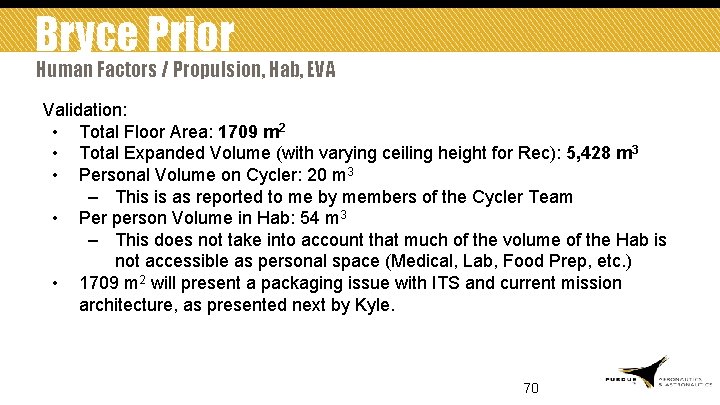
Bryce Prior Human Factors / Propulsion, Hab, EVA Validation: • Total Floor Area: 1709 m 2 • Total Expanded Volume (with varying ceiling height for Rec): 5, 428 m 3 • Personal Volume on Cycler: 20 m 3 – This is as reported to me by members of the Cycler Team • Per person Volume in Hab: 54 m 3 – This does not take into account that much of the volume of the Hab is not accessible as personal space (Medical, Lab, Food Prep, etc. ) • 1709 m 2 will present a packaging issue with ITS and current mission architecture, as presented next by Kyle. 70
![Bryce Prior Human Factors Propulsion Hab EVA 1 Shimabukuro B From the Bryce Prior Human Factors / Propulsion, Hab, EVA [1] Shimabukuro, B. , “From the](https://slidetodoc.com/presentation_image_h/184fd3da0ebff5691a469b53cfd00971/image-71.jpg)
Bryce Prior Human Factors / Propulsion, Hab, EVA [1] Shimabukuro, B. , “From the Archives: Cooking on a Submarine, ” Bremerton-Olympic Peninsula Council of the U. S. Navy League Available: https: //bremolympicnlus. wordpress. com/2013/12/17/from-the-archives-cooking-on-a-submarine/. [2] “The Greensboro, ” Modular Buildings and Mobile Offices Available: http: //www. roseoffices. com/floorplan-images/the-greensboro/. [3] “Hospital beds (per 1, 000 people), ” Hospital beds (per 1, 000 people) | Data Available: http: //data. worldbank. org/indicator/SH. MED. BEDS. ZS. [4]“Guidelines for School Facilities In Virginia’s Public Schools, ” Virginia Department of Education Available: http: //www. doe. virginia. gov/support/facility_construction/school_construction/regs_guidelines/guidelines. pdf. [5]“State Requirements for Educational Facilities, ” Florida Department of Education Available: http: //www. fldoe. org/core/fileparse. php/7738/urlt/srefrule 14. pdf. [6] "Room Layouts, " Concordia University [online]. http: //www. cune. edu/student-life/residence-hall-rooms/ [retrieved 24 January 2017]. [7] "SD Mines, " South Dakota School of Mines & Technology [online]. http: //www. sdsmt. edu/Campus-Life/Housing-and-Dining/Residence-Life/Housing-Information/Room. Dimensions/ [retrieved 24 January 2017]. [8] "University Housing, " Southern Illinois University [online]. https: //www. siue. edu/housing/woodland/room-dimensions. shtml [retrieved 24 January 2017]. [9] "Housing and Resident Life, " University of Southern Indiana [online]. https: //www. usi. edu/housing/frequently-asked-questions/moving-to-campus/room-size/ [retrieved 24 January 2017]. [10] "University Residences, " Purdue University [online]. https: //www. housing. purdue. edu/Housing/Residences/Earhart/layout. html [retrieved 24 January 2017]. [11] "University Residences, " Purdue University [online]. https: //www. housing. purdue. edu/Housing/Residences/index. html [retrieved 24 January 2017]. [12] architecture CAD “Room Sizes” [Online database] URL: http: //www 2. d 125. org/im/ACAD/pdf/room_sizes. pdf[cited 24 January 2017] [13] All about teaching English in Japan. com “Take a peek inside a “Typical” Tokyo Apatment” [online]. http: //www. all-about-teaching-english-injapan. com/Tokyoapartment. html[retrieved 24 January 2017] [ 14] The Royal Borough of Kensington and Chelsea “Houses in multiple occupation”[online]. https: //www. rbkc. gov. uk/pdf/HMO%20 standards%20 hostels%20 and%20 staff%20 accommodation. pdf [retrieved 24 January 2017] [15] New York city government “Chapter 2 housing maintenance code” New York city RGB [online]. http: //www. nycrgb. org/html/resources/hmc/sub 3/art 4. html [retrieved 24 January 2017]. [16] House Plans Helper “Bedroom Size” [Online database] URL: http: //www. houseplanshelper. com/bedroom-size. html[cited 24 January 2017] 71[Retrieved 23 January 2017]. [17] “Module List - International Space Station, ” Google Sites Available: https: //sites. google. com/site/issinspire/home/design/modules
![Bryce Prior Human Factors Propulsion Hab EVA 15 New York city government Chapter Bryce Prior Human Factors / Propulsion, Hab, EVA [15] New York city government “Chapter](https://slidetodoc.com/presentation_image_h/184fd3da0ebff5691a469b53cfd00971/image-72.jpg)
Bryce Prior Human Factors / Propulsion, Hab, EVA [15] New York city government “Chapter 2 housing maintenance code” New York city RGB [online]. http: //www. nycrgb. org/html/resources/hmc/sub 3/art 4. html [retrieved 24 January 2017]. [16] House Plans Helper “Bedroom Size” [Online database] URL: http: //www. houseplanshelper. com/bedroom-size. html[cited 24 January 2017] [17] “Module List - International Space Station, ” Google Sites Available: https: //sites. google. com/site/issinspire/home/design/modules [Retrieved 23 January 2017]. [18] E. , “Columbus , ” European Space Agency Available: http: //www. esa. int/Our_Activities/Human_Spaceflight/Columbus/European_Columbus_laboratory [Retrieved 23 January 2017]. [19] Garcia, M. , ed. , “Facts and Figures, ” NASA Available: https: //www. nasa. gov/feature/facts-and-figures [Retrieved 23 January 2017]. [20] “Kibo Laboratory, ” NASA Available: https: //www. nasa. gov/mission_pages/station/structure/elements/jem. html#. WIb. Ctxsr. JPY [Retrieved 23 January 2017]. [21] Loff, S. , ed. , “Destiny Laboratory Attached to International Space Station, ” NASA Available: https: //www. nasa. gov/content/destiny-laboratory-attached-to-internationalspace-station [Retrieved 23 January 2017]. [22] “Toilet facilities - what should workplaces have? ” http: //www. ohsrep. org. au/faqs/workplace-and-amenities/toilet-facilities-what-should-workplaces-have [Retrieved 22 January 2017]. [23] “The ADA-Compliant Restroom, ” http: //www. buildings. com/article-details/articleid/9242/title/the-ada-compliant-restroom [Retrieved 22 January 2017]. [24] Trappe, S. , Costill, D. , Gallagher, P. , Creer, A. , Peters, J. R. , Evans, H. , Riley, D. A. , and Fitts, R. H. , “Exercise in space: human skeletal muscle after 6 months aboard the International Space Station, ” Journal of Applied Physiology, vol. 106, Jan. 2009, pp. 1159– 1168. [25] “University of Wisconsin - Green Bay, ” Inside UWGreen Bay News Available: https: //news. uwgb. edu/log-news/11/03/one-million-kress-events-center-nears-usermilestone/. [Retrieved 22 January 2017]. [26] “University of Wisconsin - Green Bay, ” Enrollment - Office of Institutional Research & Assessment - University of Wisconsin - Green Bay Available: http: //www. uwgb. edu/oira/enrollment/. [Retrieved 22 January 2017]. 72
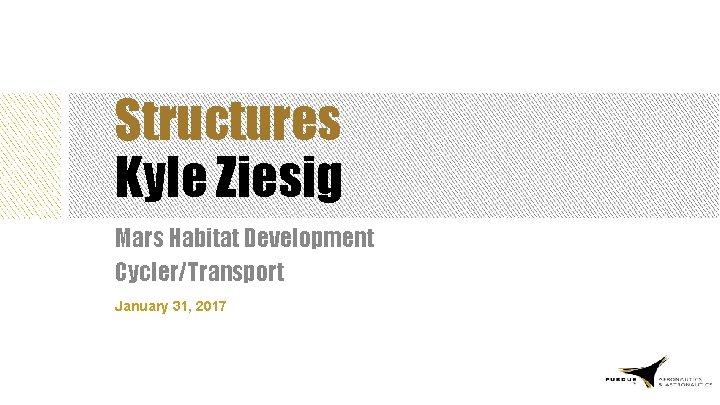
Structures Kyle Ziesig Mars Habitat Development Cycler/Transport January 31, 2017
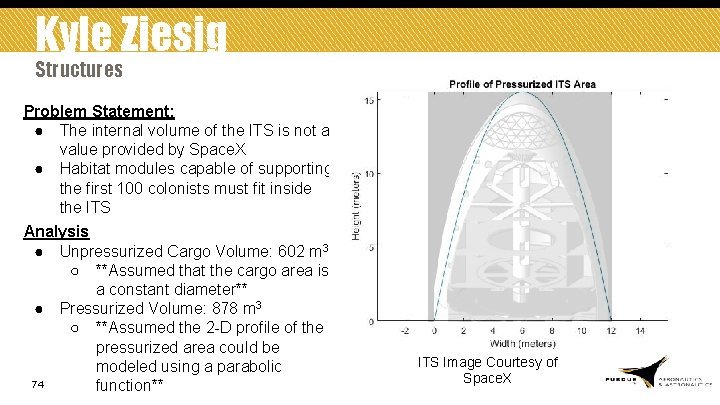
Kyle Ziesig Structures Problem Statement: ● The internal volume of the ITS is not a value provided by Space. X ● Habitat modules capable of supporting the first 100 colonists must fit inside the ITS Analysis ● Unpressurized Cargo Volume: 602 m 3 ○ **Assumed that the cargo area is a constant diameter** ● Pressurized Volume: 878 m 3 ○ **Assumed the 2 -D profile of the pressurized area could be modeled using a parabolic 74 function** ITS Image Courtesy of Space. X
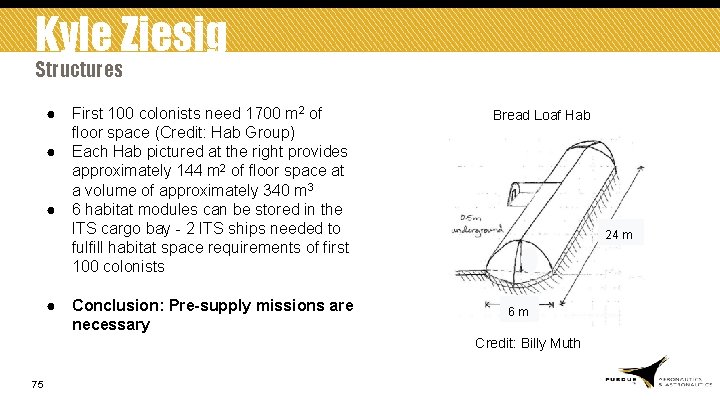
Kyle Ziesig Structures ● First 100 colonists need 1700 m 2 of floor space (Credit: Hab Group) ● Each Hab pictured at the right provides approximately 144 m 2 of floor space at a volume of approximately 340 m 3 ● 6 habitat modules can be stored in the ITS cargo bay - 2 ITS ships needed to fulfill habitat space requirements of first 100 colonists ● Conclusion: Pre-supply missions are necessary Bread Loaf Hab 24 m 6 m Credit: Billy Muth 75
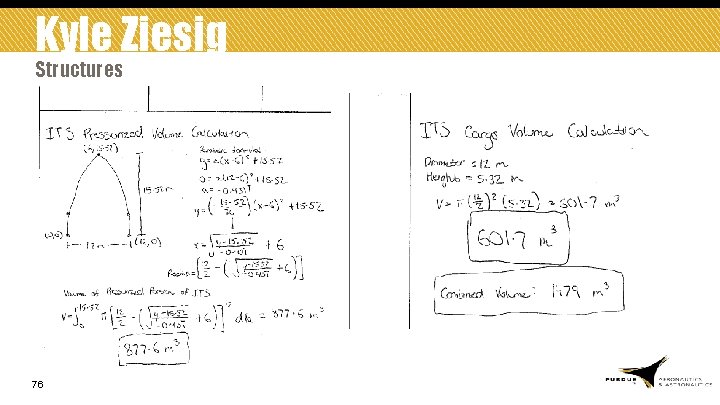
Kyle Ziesig Structures 76
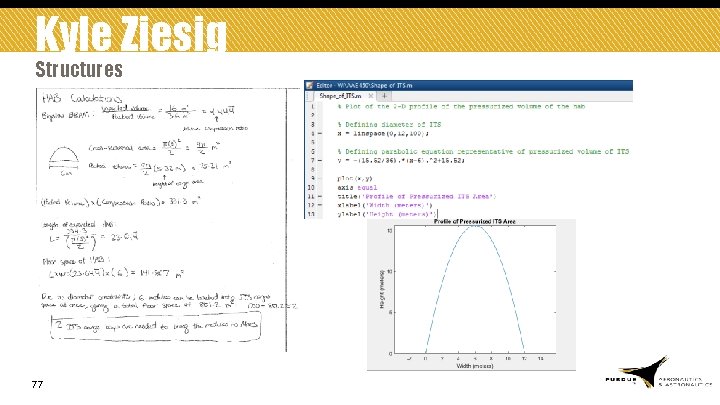
Kyle Ziesig Structures 77
![Kyle Ziesig Structures References 1 Space X Space X Available http www spacex Kyle Ziesig Structures References [1] “Space. X, ” Space. X Available: http: //www. spacex.](https://slidetodoc.com/presentation_image_h/184fd3da0ebff5691a469b53cfd00971/image-78.jpg)
Kyle Ziesig Structures References [1] “Space. X, ” Space. X Available: http: //www. spacex. com/sites/spacex/files/mars_presentation. pdf [Retrieved 22 January 2017]. [2] “BEAM: The Experimental Platform, ” Bigelow Aerospace Available: http: //www. bigelowaerospace. com/beam/. 78
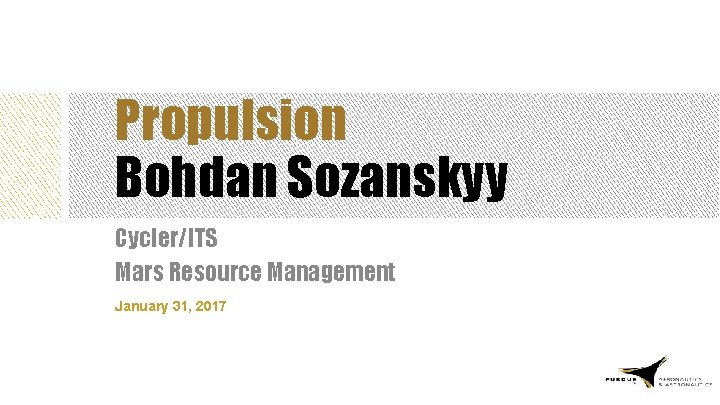
Propulsion Bohdan Sozanskyy Cycler/ITS Mars Resource Management January 31, 2017
![Bohdan Sozanksyy Propulsion Problem Producing fuel for return trip LOXMethane ITS Propellant 1 Bohdan Sozanksyy Propulsion */ Problem: Producing fuel for return trip (LOX/Methane) ITS Propellant [1]:](https://slidetodoc.com/presentation_image_h/184fd3da0ebff5691a469b53cfd00971/image-80.jpg)
Bohdan Sozanksyy Propulsion */ Problem: Producing fuel for return trip (LOX/Methane) ITS Propellant [1]: O/F Ratio[3] 3. 8 O 2 CH 4 1544 Mg 406. 3 Mg Total 1950 Mg Using Sabatier/Electrolysis - Reverse Water Gas Shift Reaction (S/E - RWGS) ● Requires H 2 source: Earth or Mars ○ Need 101. 6 Mg H 2 to refuel 1 ITS (20: 1 propellant conversion leverage) ○ Potentially use electrolysis to convert H 2 O to H 2 (would need 914. 4 Mg) ● Produces propellant at O/F of 4. 0 (excess O 2 81. 2 Mg) 80
![Bohdan Sozanksyy Propulsion Reactor Unit Size Estimate 2 Producing 500 kgday of Bohdan Sozanksyy Propulsion */ Reactor Unit Size Estimate [2] ● Producing 500 kg/day of](https://slidetodoc.com/presentation_image_h/184fd3da0ebff5691a469b53cfd00971/image-81.jpg)
Bohdan Sozanksyy Propulsion */ Reactor Unit Size Estimate [2] ● Producing 500 kg/day of propellant (CH 4 + O 2 ) ● Mass: 980 kg ● Power: 279. 2 k. W Need about 5 to produce required fuel ● Mass: 4900 kg (4. 9 Mg) ● Power: 1, 395 k. W ● Note: does not scale linearly, more efficient with increased size 81 Approximate Production Rates Needed for Synodic Period per ITS Resource Mg/24 hrs H 2 O 1. 28 H 2 0. 13 CH 4 0. 52 O 2 1. 97
![Bohdan Sozanksyy Propulsion References 1 Richardson D Elon Musk shows off Interplanetary Bohdan Sozanksyy Propulsion */ References [1] Richardson, D. , “Elon Musk shows off Interplanetary](https://slidetodoc.com/presentation_image_h/184fd3da0ebff5691a469b53cfd00971/image-82.jpg)
Bohdan Sozanksyy Propulsion */ References [1] Richardson, D. , “Elon Musk shows off Interplanetary Transport System, ” Spaceflight Insider. Available: http: //www. spaceflightinsider. com/organizations/space-exploration-technologies/elon-musk-shows-off-interplanetary-transportsystem/ [2] Robert Zubrin, Brian Frankie, Tomoko Kito, Robert Zubrin, Brian Frankie, and Tomoko Kito. "Mars in-situ resource utilization based on the reverse water gas shift - Experiments and mission applications", 33 rd Joint Propulsion Conference and Exhibit, Joint Propulsion Conferences, () http: //dx. doi. org/10. 2514/6. 1997 -2767 [3] Urban, T. , “How (and Why) Space. X Will Colonize Mars - Page 5 of 5, ”Wait But Why. Available: http: //waitbutwhy. com/2015/08/how-and-why-spacex-will-colonize-mars. html/5. [4]“Synthesizing Fuel: Methane for Nothing and the Oxygen is Free? , ”The Sabatier Process - Part 2 Available: http: //www. digipac. ca/chemical/mtom/contents/chapter 3/sabatier 2. htm. [5]Muscatello, A. C. , and Santiago-Maldonado, E. , “Mars In Situ Resource Utilization Technology Evaluation, ”NASAAvailable: https: //ntrs. nasa. gov/archive/nasa/casi. ntrs. nasa. gov/20120001775. pdf. 82
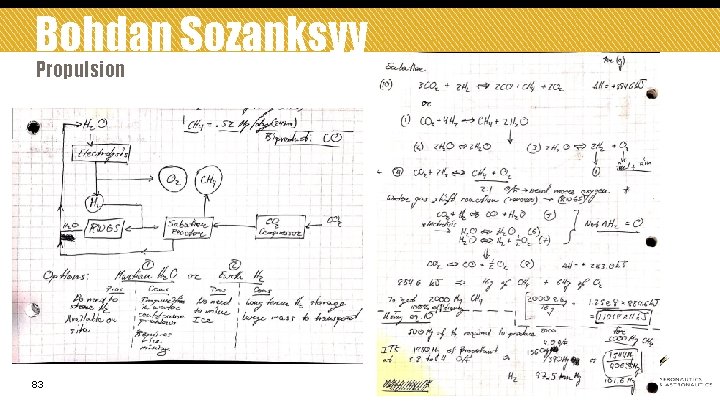
Bohdan Sozanksyy Propulsion */ 83
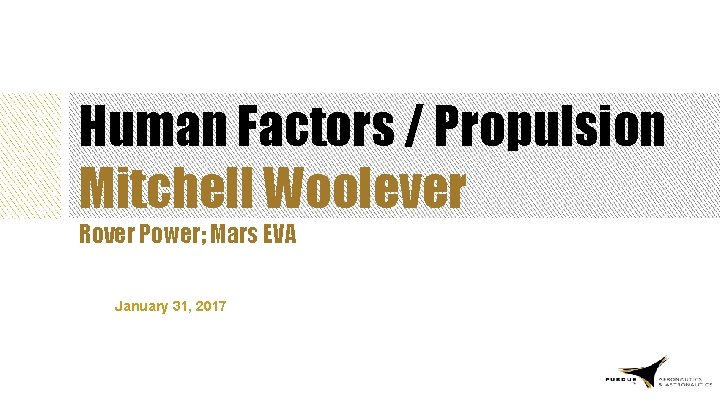
Human Factors / Propulsion Mitchell Woolever Rover Power; Mars EVA January 31, 2017
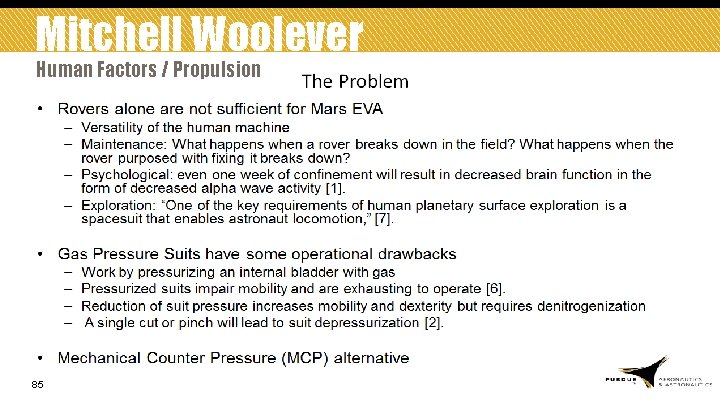
Mitchell Woolever Human Factors / Propulsion 85
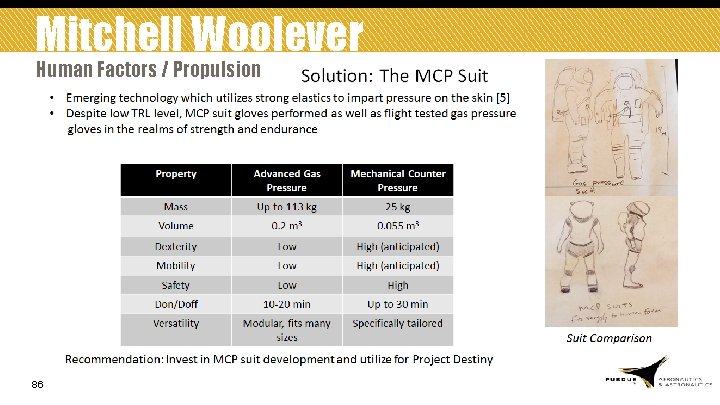
Mitchell Woolever Human Factors / Propulsion 86
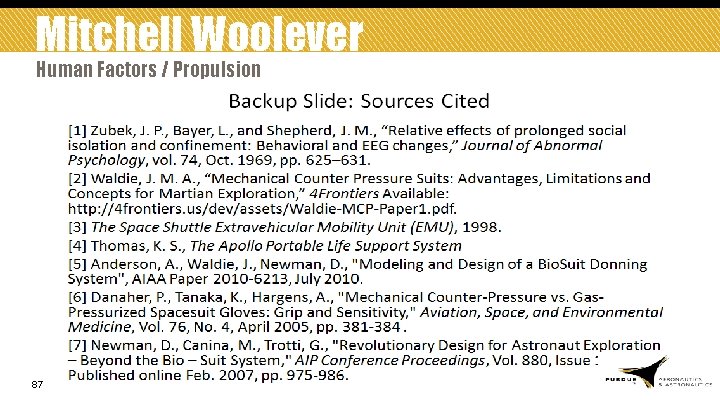
Mitchell Woolever Human Factors / Propulsion 87
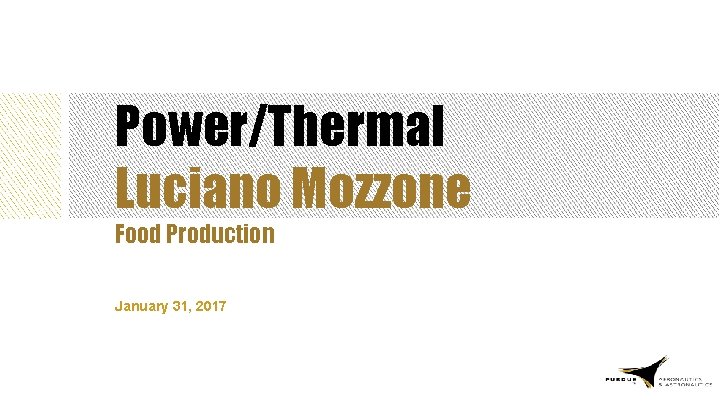
Power/Thermal Luciano Mozzone Food Production January 31, 2017
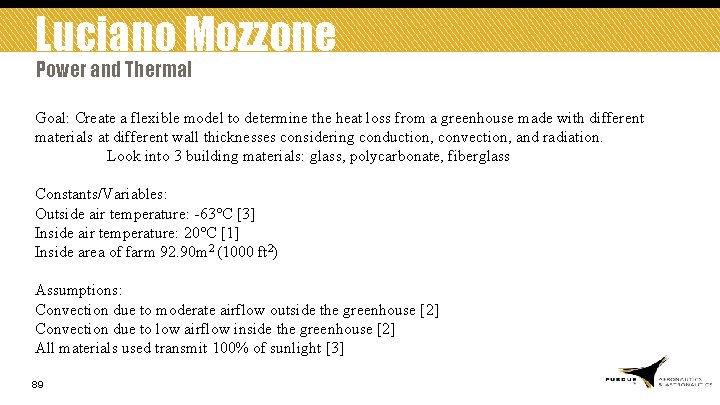
Luciano Mozzone Power and Thermal Goal: Create a flexible model to determine the heat loss from a greenhouse made with different materials at different wall thicknesses considering conduction, convection, and radiation. Look into 3 building materials: glass, polycarbonate, fiberglass Constants/Variables: Outside air temperature: -63°C [3] Inside air temperature: 20°C [1] Inside area of farm 92. 90 m 2 (1000 ft 2) Assumptions: Convection due to moderate airflow outside the greenhouse [2] Convection due to low airflow inside the greenhouse [2] All materials used transmit 100% of sunlight [3] 89
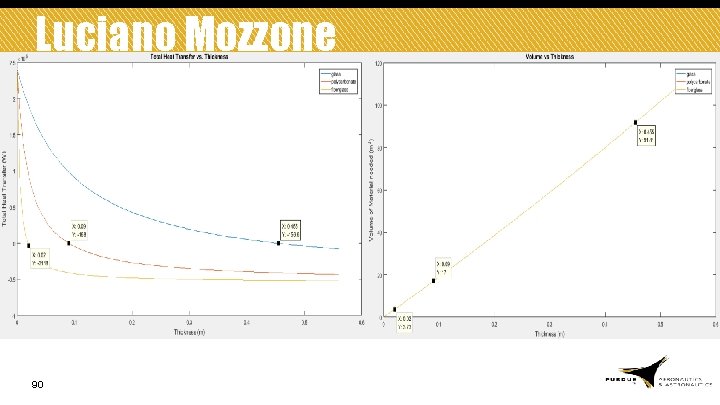
Luciano Mozzone Power and Thermal 90
![Luciano Mozzone Power and Thermal Backup Slide Resources 1 Iga J L Iga Luciano Mozzone Power and Thermal Backup Slide: Resources [1] Iga, J. L. , Iga,](https://slidetodoc.com/presentation_image_h/184fd3da0ebff5691a469b53cfd00971/image-91.jpg)
Luciano Mozzone Power and Thermal Backup Slide: Resources [1] Iga, J. L. , Iga, C. L. , and Flores, R. A. , “Effect of air density variations on greenhouse temperature model, ” Mathematical and Computer Modelling, vol. 47, 2008, pp. 855– 867. [2] “Convective Heat Transfer Coefficients Table Chart, ” Engineers Edge - Reference Data for Engineers Available: http: //www. engineersedge. com/heat_transfer/convective_heat_transfer_coefficients__13378. htm [3] Williams, D. R. , “Mars Fact Sheet, ” NASA Available: http: //nssdc. gsfc. nasa. gov/planetary/factsheet/marsfact. html. 91
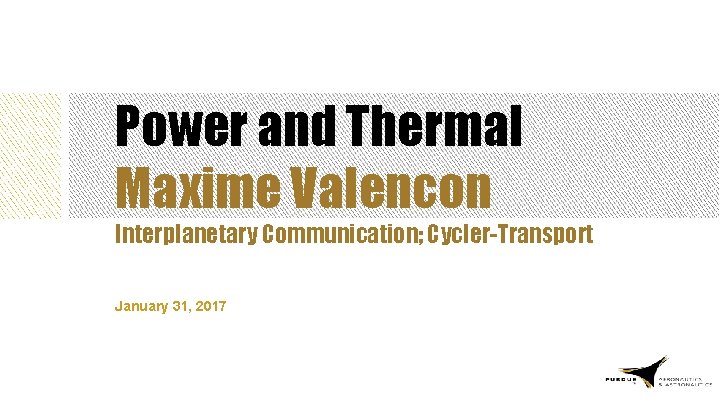
Power and Thermal Maxime Valencon Interplanetary Communication; Cycler-Transport January 31, 2017
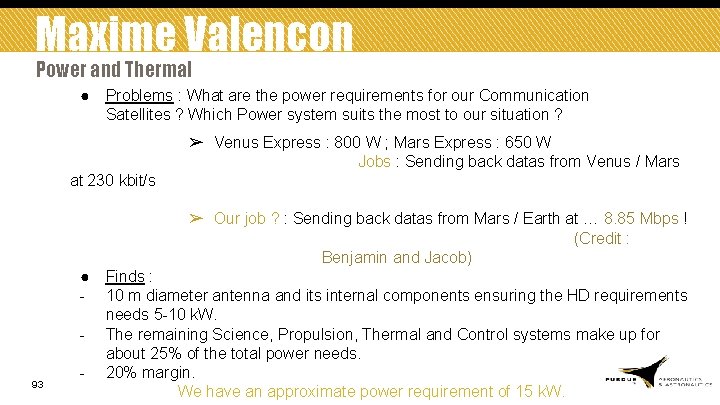
Maxime Valencon Power and Thermal ● Problems : What are the power requirements for our Communication Satellites ? Which Power system suits the most to our situation ? ➢ Venus Express : 800 W ; Mars Express : 650 W Jobs : Sending back datas from Venus / Mars at 230 kbit/s ➢ Our job ? : Sending back datas from Mars / Earth at … 8. 85 Mbps ! (Credit : Benjamin and Jacob) 93 ● Finds : - 10 m diameter antenna and its internal components ensuring the HD requirements needs 5 -10 k. W. - The remaining Science, Propulsion, Thermal and Control systems make up for about 25% of the total power needs. - 20% margin. We have an approximate power requirement of 15 k. W.
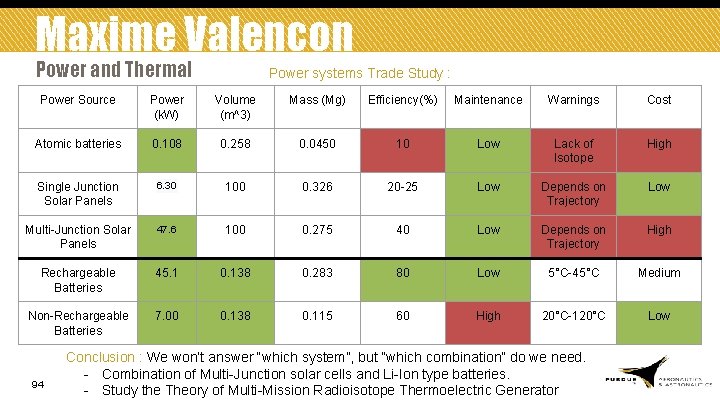
Maxime Valencon Power and Thermal Power systems Trade Study : Power Source Power (k. W) Volume (m^3) Mass (Mg) Efficiency(%) Maintenance Warnings Cost Atomic batteries 0. 108 0. 258 0. 0450 10 Low Lack of Isotope High Single Junction Solar Panels 6. 30 100 0. 326 20 -25 Low Depends on Trajectory Low Multi-Junction Solar Panels 47. 6 100 0. 275 40 Low Depends on Trajectory High Rechargeable Batteries 45. 1 0. 138 0. 283 80 Low 5°C-45°C Medium Non-Rechargeable Batteries 7. 00 0. 138 0. 115 60 High 20°C-120°C Low 94 Conclusion : We won’t answer “which system”, but “which combination” do we need. - Combination of Multi-Junction solar cells and Li-Ion type batteries. - Study the Theory of Multi-Mission Radioisotope Thermoelectric Generator
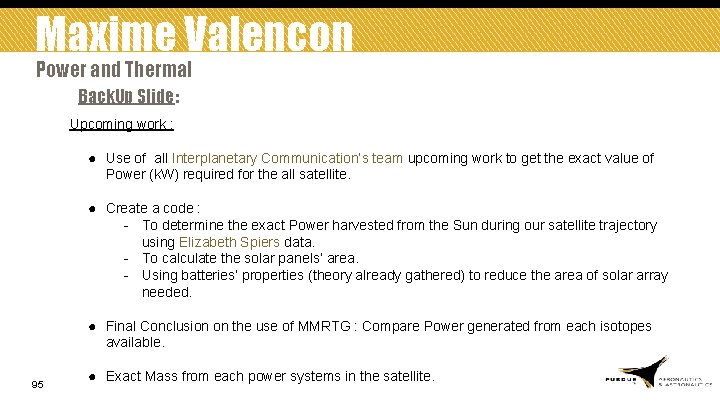
Maxime Valencon Power and Thermal Back. Up Slide: Upcoming work : ● Use of all Interplanetary Communication’s team upcoming work to get the exact value of Power (k. W) required for the all satellite. ● Create a code : - To determine the exact Power harvested from the Sun during our satellite trajectory using Elizabeth Spiers data. - To calculate the solar panels’ area. - Using batteries’ properties (theory already gathered) to reduce the area of solar array needed. ● Final Conclusion on the use of MMRTG : Compare Power generated from each isotopes available. 95 ● Exact Mass from each power systems in the satellite.
![Maxime Valencon Power and Thermal References 96 1 J Arenberg J Flynn A Maxime Valencon Power and Thermal References: 96 [1] J. Arenberg, J. Flynn , A.](https://slidetodoc.com/presentation_image_h/184fd3da0ebff5691a469b53cfd00971/image-96.jpg)
Maxime Valencon Power and Thermal References: 96 [1] J. Arenberg, J. Flynn , A. Cohen, R. Lynch and J. Cooper, “Status of the JWST Sunshield and Spacecraft”, 08/13/2016, [retrieved 01/19/2017] [2] Scott W. Benson, “Solar Power for Outer Planets Study”, 11/08/2007, [retrieved 01/20/2017] [3] Natalya V. Yastrebova “High-efficiency multi-junction solar cells: Current status and future potential ”, 2007, [retrieved 01/22/2017] [4] Barbara Mc. Kissock, Patricia Loyselle, and Elisa Vogel, “Guidelines on Lithium-ion Battery Use in Space Applications”, May 2009, [retrieved 01/21/2017] [5] “Rechargeable Li-ion battery systems Light energy storage for space applications”, October 2006, [retrieved 01/26/2017] [6] Suhas Kumar, “Atomic Batteries: Energy from Radioactivity”, 11/17/2015, [retrieved 01/26/2017] [7] Gary L. Bennett, “Space Nuclear Power: Opening the Final Frontier”, 2006, [retrieved 01/27/2017] [8] “Venus Express : Data sheet”, URL : http: //sci. esa. int/venus-express/33011 -fact-sheet/, [retrieved 01/26/2017]
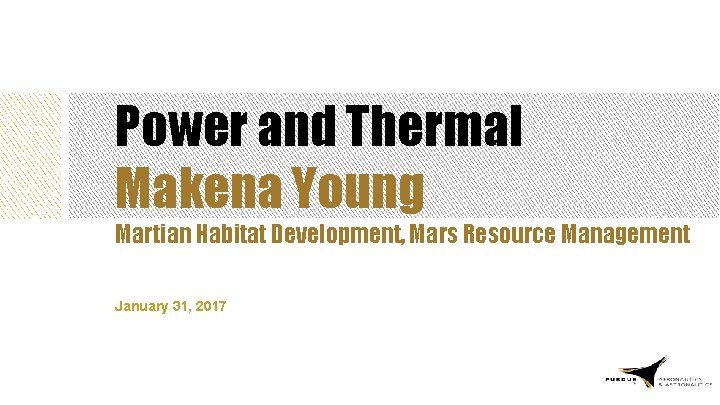
Power and Thermal Makena Young Martian Habitat Development, Mars Resource Management January 31, 2017
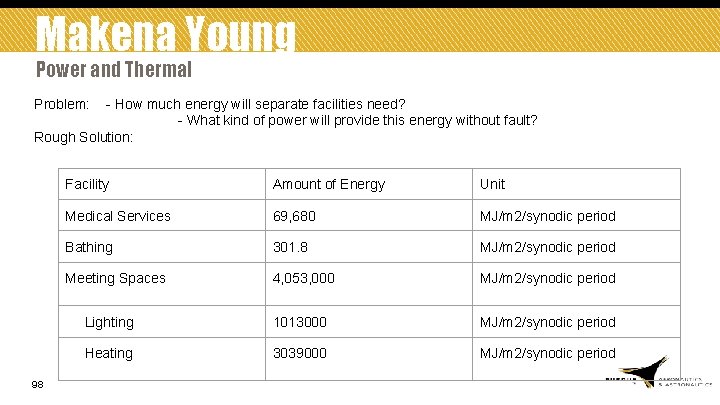
Makena Young Power and Thermal Problem: - How much energy will separate facilities need? - What kind of power will provide this energy without fault? Rough Solution: 98 Facility Amount of Energy Unit Medical Services 69, 680 MJ/m 2/synodic period Bathing 301. 8 MJ/m 2/synodic period Meeting Spaces 4, 053, 000 MJ/m 2/synodic period Lighting 1013000 MJ/m 2/synodic period Heating 3039000 MJ/m 2/synodic period
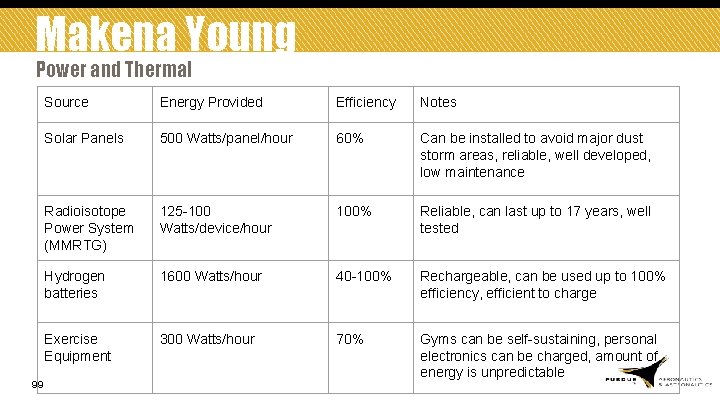
Makena Young Power and Thermal 99 Source Energy Provided Efficiency Notes Solar Panels 500 Watts/panel/hour 60% Can be installed to avoid major dust storm areas, reliable, well developed, low maintenance Radioisotope Power System (MMRTG) 125 -100 Watts/device/hour 100% Reliable, can last up to 17 years, well tested Hydrogen batteries 1600 Watts/hour 40 -100% Rechargeable, can be used up to 100% efficiency, efficient to charge Exercise Equipment 300 Watts/hour 70% Gyms can be self-sustaining, personal electronics can be charged, amount of energy is unpredictable
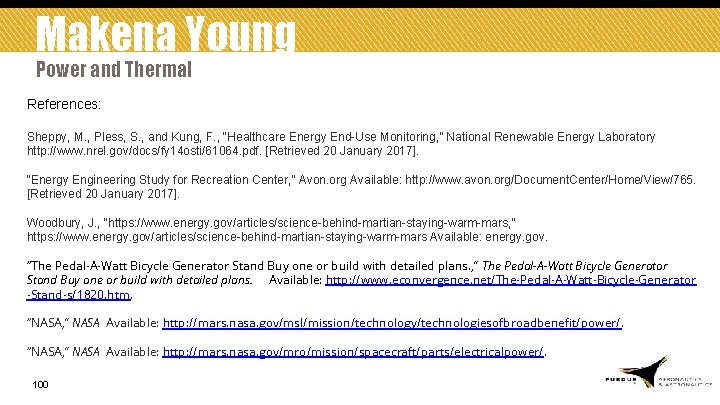
Makena Young Power and Thermal References: Sheppy, M. , Pless, S. , and Kung, F. , “Healthcare Energy End-Use Monitoring, ” National Renewable Energy Laboratory http: //www. nrel. gov/docs/fy 14 osti/61064. pdf. [Retrieved 20 January 2017]. “Energy Engineering Study for Recreation Center, ” Avon. org Available: http: //www. avon. org/Document. Center/Home/View/765. [Retrieved 20 January 2017]. Woodbury, J. , “https: //www. energy. gov/articles/science-behind-martian-staying-warm-mars, ” https: //www. energy. gov/articles/science-behind-martian-staying-warm-mars Available: energy. gov. “The Pedal-A-Watt Bicycle Generator Stand Buy one or build with detailed plans. , ” The Pedal-A-Watt Bicycle Generator Stand Buy one or build with detailed plans. Available: http: //www. econvergence. net/The-Pedal-A-Watt-Bicycle-Generator -Stand-s/1820. htm. “NASA, ” NASA Available: http: //mars. nasa. gov/msl/mission/technology/technologiesofbroadbenefit/power/. “NASA, ” NASA Available: http: //mars. nasa. gov/mro/mission/spacecraft/parts/electricalpower/. 100
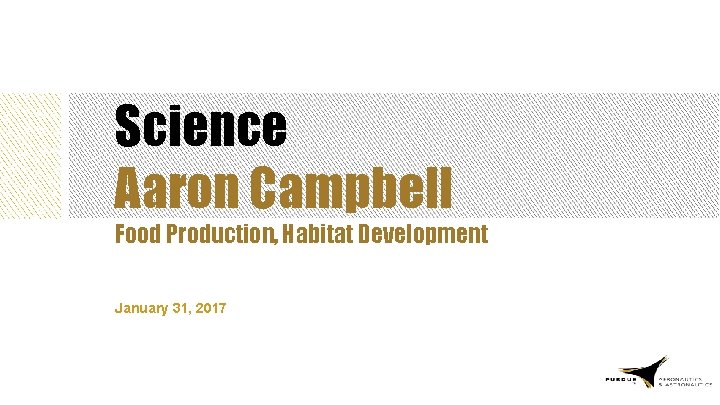
Science Aaron Campbell Food Production, Habitat Development January 31, 2017
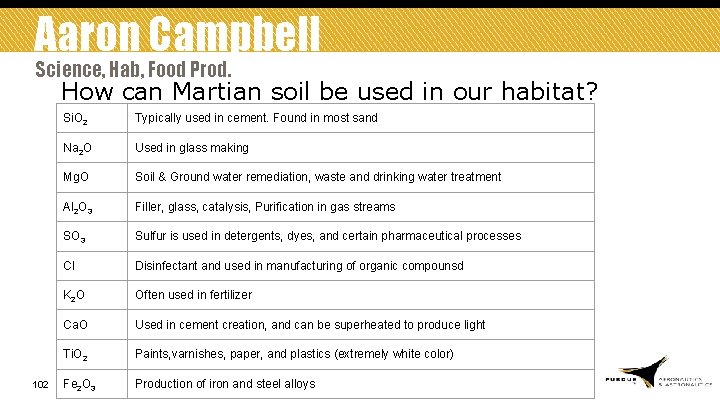
Aaron Campbell Science, Hab, Food Prod. How can Martian soil be used in our habitat? 102 Si. O 2 Typically used in cement. Found in most sand Na 2 O Used in glass making Mg. O Soil & Ground water remediation, waste and drinking water treatment Al 2 O 3 Filler, glass, catalysis, Purification in gas streams SO 3 Sulfur is used in detergents, dyes, and certain pharmaceutical processes Cl Disinfectant and used in manufacturing of organic compounsd K 2 O Often used in fertilizer Ca. O Used in cement creation, and can be superheated to produce light Ti. O 2 Paints, varnishes, paper, and plastics (extremely white color) Fe 2 O 3 Production of iron and steel alloys
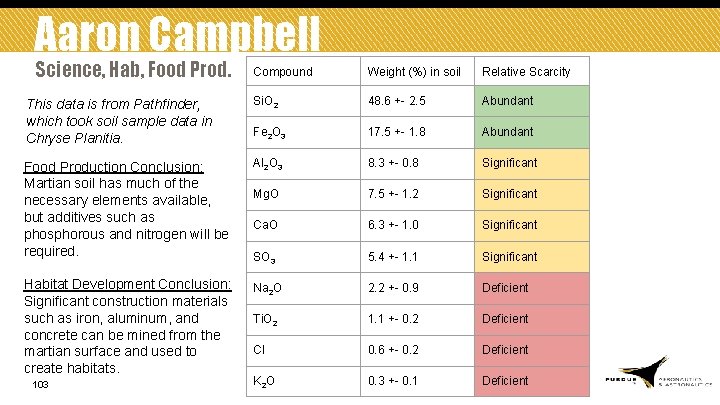
Aaron Campbell Science, Hab, Food Prod. Compound Weight (%) in soil Relative Scarcity This data is from Pathfinder, which took soil sample data in Chryse Planitia. Si. O 2 48. 6 +- 2. 5 Abundant Fe 2 O 3 17. 5 +- 1. 8 Abundant Food Production Conclusion: Martian soil has much of the necessary elements available, but additives such as phosphorous and nitrogen will be required. Al 2 O 3 8. 3 +- 0. 8 Significant Mg. O 7. 5 +- 1. 2 Significant Ca. O 6. 3 +- 1. 0 Significant SO 3 5. 4 +- 1. 1 Significant Habitat Development Conclusion: Significant construction materials such as iron, aluminum, and concrete can be mined from the martian surface and used to create habitats. Na 2 O 2. 2 +- 0. 9 Deficient Ti. O 2 1. 1 +- 0. 2 Deficient Cl 0. 6 +- 0. 2 Deficient K 2 O 0. 3 +- 0. 1 Deficient 103
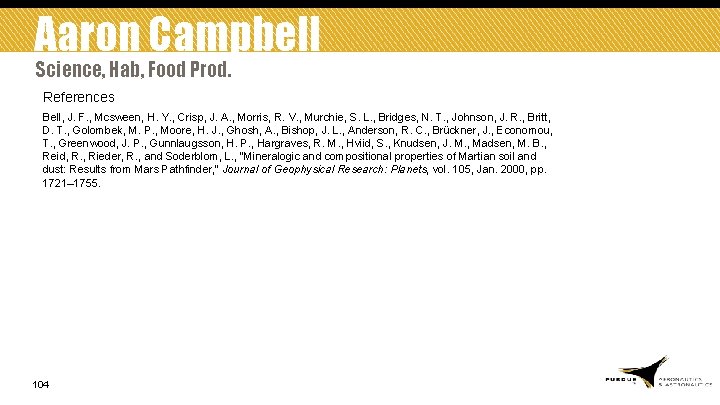
Aaron Campbell Science, Hab, Food Prod. References Bell, J. F. , Mcsween, H. Y. , Crisp, J. A. , Morris, R. V. , Murchie, S. L. , Bridges, N. T. , Johnson, J. R. , Britt, D. T. , Golombek, M. P. , Moore, H. J. , Ghosh, A. , Bishop, J. L. , Anderson, R. C. , Brückner, J. , Economou, T. , Greenwood, J. P. , Gunnlaugsson, H. P. , Hargraves, R. M. , Hviid, S. , Knudsen, J. M. , Madsen, M. B. , Reid, R. , Rieder, R. , and Soderblom, L. , “Mineralogic and compositional properties of Martian soil and dust: Results from Mars Pathfinder, ” Journal of Geophysical Research: Planets, vol. 105, Jan. 2000, pp. 1721– 1755. 104
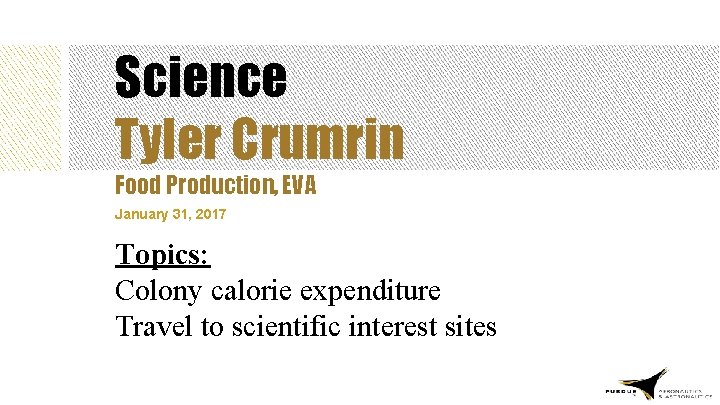
Science Tyler Crumrin Food Production, EVA January 31, 2017 Topics: Colony calorie expenditure Travel to scientific interest sites
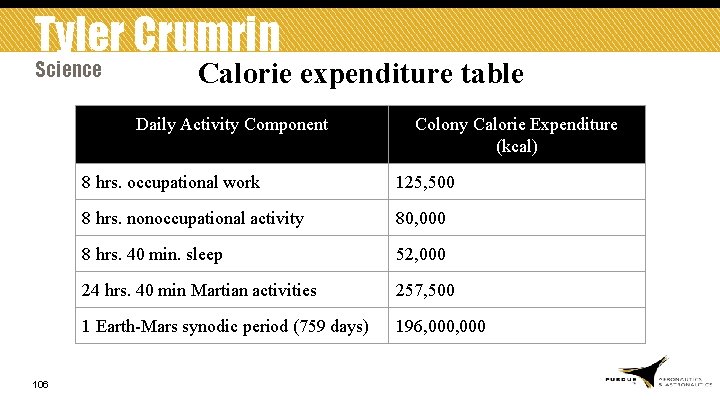
Tyler Crumrin Science Calorie expenditure table Daily Activity Component 106 Colony Calorie Expenditure (kcal) 8 hrs. occupational work 125, 500 8 hrs. nonoccupational activity 80, 000 8 hrs. 40 min. sleep 52, 000 24 hrs. 40 min Martian activities 257, 500 1 Earth-Mars synodic period (759 days) 196, 000
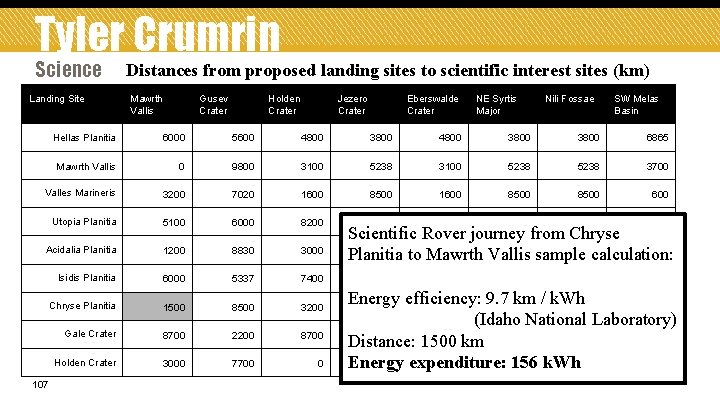
Tyler Crumrin Science Landing Site Distances from proposed landing sites to scientific interest sites (km) Mawrth Vallis Gusev Crater Holden Crater Jezero Crater Eberswalde Crater NE Syrtis Major Nili Fossae SW Melas Basin Hellas Planitia 6000 5600 4800 3800 6865 Mawrth Vallis 0 9800 3100 5238 3700 Valles Marineris 3200 7020 1600 8500 600 Utopia Planitia 5100 6000 8200 2300 8400 Acidalia Planitia 1200 8830 3000 Isidis Planitia 6000 5337 7400 700 700 9500 Chryse Planitia 1500 8500 3200 Gale Crater 8700 2200 8700 Holden Crater 3000 7700 0 107 Scientific Rover journey from Chryse 6700 3000 6700 2900 Planitia to Mawrth Vallis sample 6700 calculation: Energy efficiency: 9. 76200 km / k. Wh 6200 3200 2900 (Idaho National Laboratory) 3800 8700 Distance: 1500 km 3800 7200 100 7200 8700 Energy expenditure: 156 k. Wh 7200
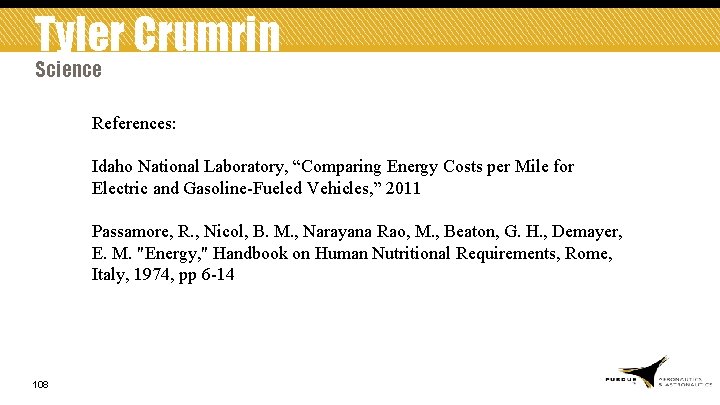
Tyler Crumrin Science References: Idaho National Laboratory, “Comparing Energy Costs per Mile for Electric and Gasoline-Fueled Vehicles, ” 2011 Passamore, R. , Nicol, B. M. , Narayana Rao, M. , Beaton, G. H. , Demayer, E. M. "Energy, " Handbook on Human Nutritional Requirements, Rome, Italy, 1974, pp 6 -14 108
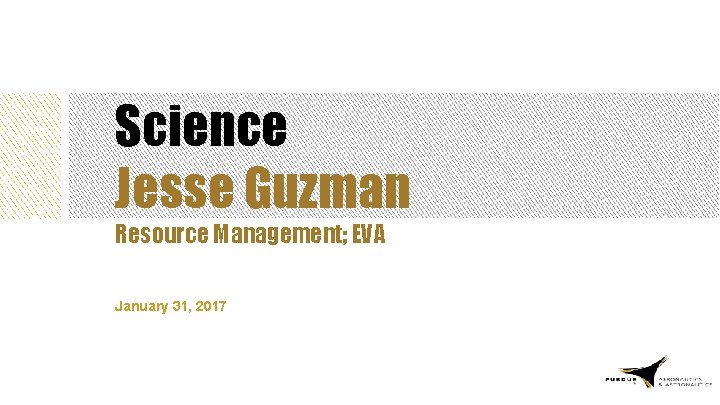
Science Jesse Guzman Resource Management; EVA January 31, 2017
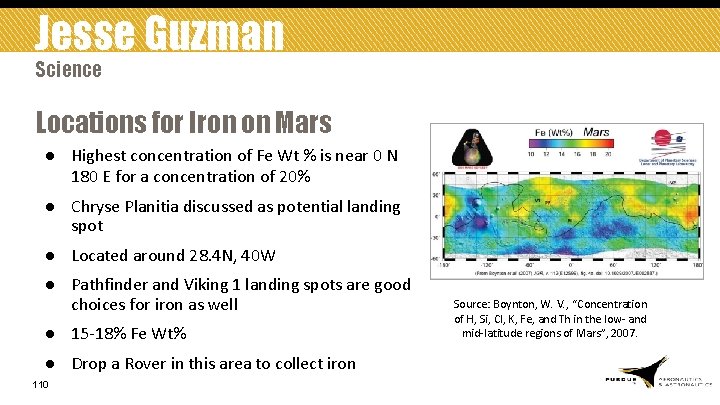
Jesse Guzman Science Locations for Iron on Mars ● Highest concentration of Fe Wt % is near 0 N 180 E for a concentration of 20% ● Chryse Planitia discussed as potential landing spot ● Located around 28. 4 N, 40 W ● Pathfinder and Viking 1 landing spots are good choices for iron as well ● 15 -18% Fe Wt% ● Drop a Rover in this area to collect iron 110 Source: Boynton, W. V. , “Concentration of H, Si, Cl, K, Fe, and Th in the low- and mid-latitude regions of Mars”, 2007.
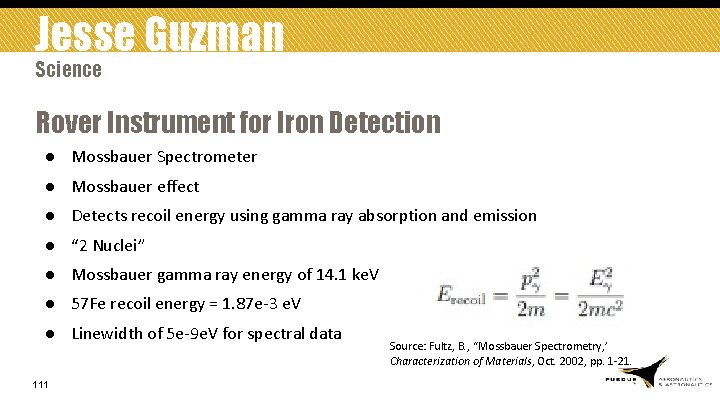
Jesse Guzman Science Rover Instrument for Iron Detection ● Mossbauer Spectrometer ● Mossbauer effect ● Detects recoil energy using gamma ray absorption and emission ● “ 2 Nuclei” ● Mossbauer gamma ray energy of 14. 1 ke. V ● 57 Fe recoil energy = 1. 87 e-3 e. V ● Linewidth of 5 e-9 e. V for spectral data 111 Source: Fultz, B. , “Mossbauer Spectrometry, ’ Characterization of Materials, Oct. 2002, pp. 1 -21.
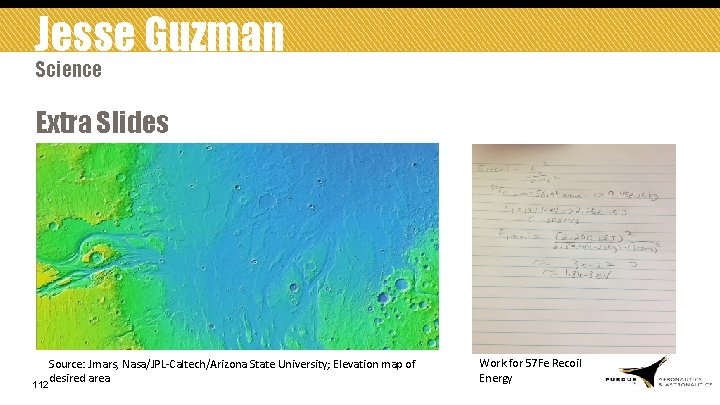
Jesse Guzman Science Extra Slides Source: Jmars, Nasa/JPL-Caltech/Arizona State University; Elevation map of desired area 112 Work for 57 Fe Recoil Energy
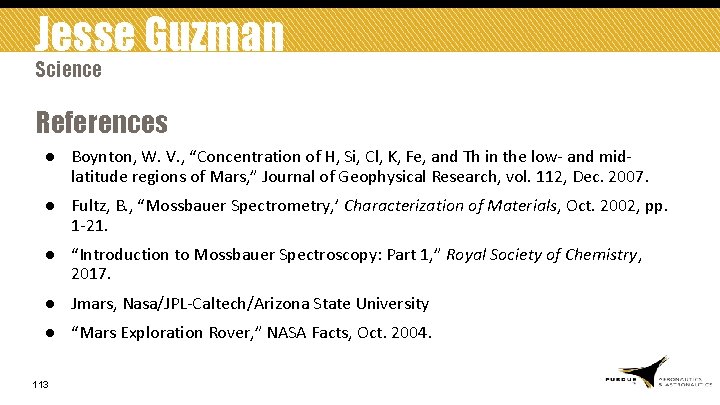
Jesse Guzman Science References ● Boynton, W. V. , “Concentration of H, Si, Cl, K, Fe, and Th in the low- and midlatitude regions of Mars, ” Journal of Geophysical Research, vol. 112, Dec. 2007. ● Fultz, B. , “Mossbauer Spectrometry, ’ Characterization of Materials, Oct. 2002, pp. 1 -21. ● “Introduction to Mossbauer Spectroscopy: Part 1, ” Royal Society of Chemistry, 2017. ● Jmars, Nasa/JPL-Caltech/Arizona State University ● “Mars Exploration Rover, ” NASA Facts, Oct. 2004. 113
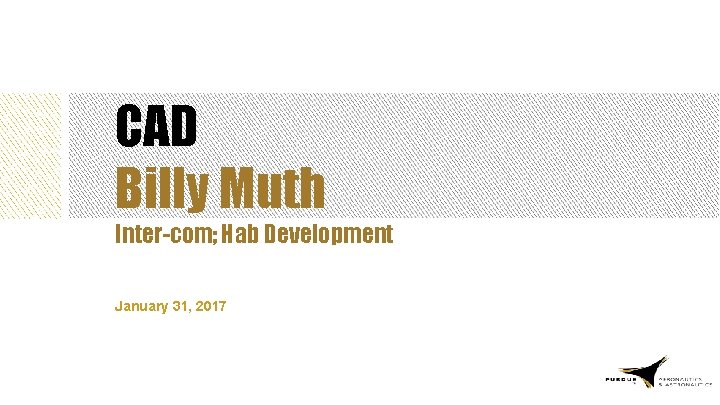
CAD Billy Muth Inter-com; Hab Development January 31, 2017
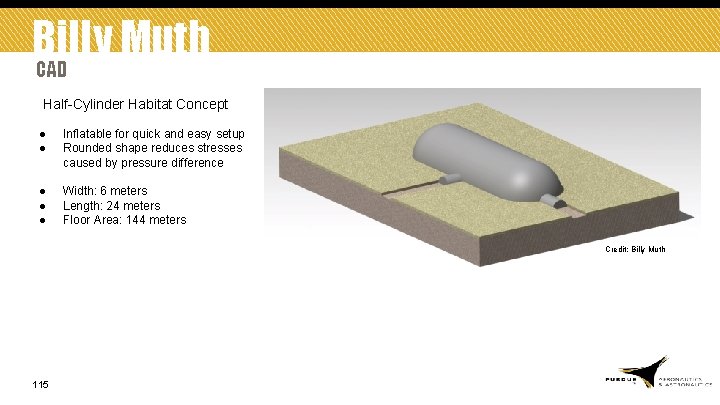
Billy Muth CAD Half-Cylinder Habitat Concept ● ● Inflatable for quick and easy setup Rounded shape reduces stresses caused by pressure difference ● ● ● Width: 6 meters Length: 24 meters Floor Area: 144 meters Credit: Billy Muth 115
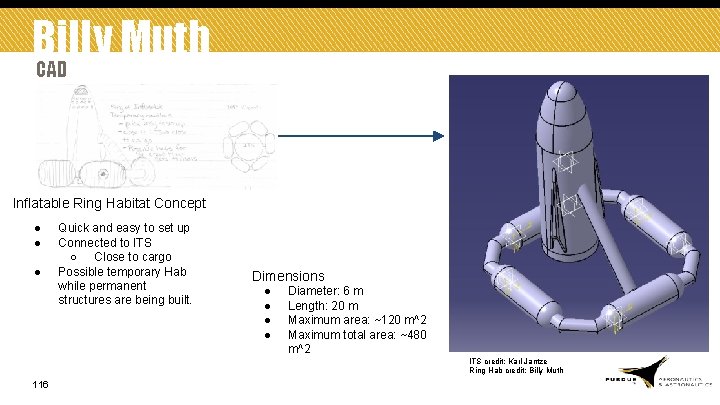
Billy Muth CAD Inflatable Ring Habitat Concept ● ● ● Quick and easy to set up Connected to ITS ○ Close to cargo Possible temporary Hab while permanent structures are being built. Dimensions ● ● Diameter: 6 m Length: 20 m Maximum area: ~120 m^2 Maximum total area: ~480 m^2 ITS credit: Karl Jantze Ring Hab credit: Billy Muth 116
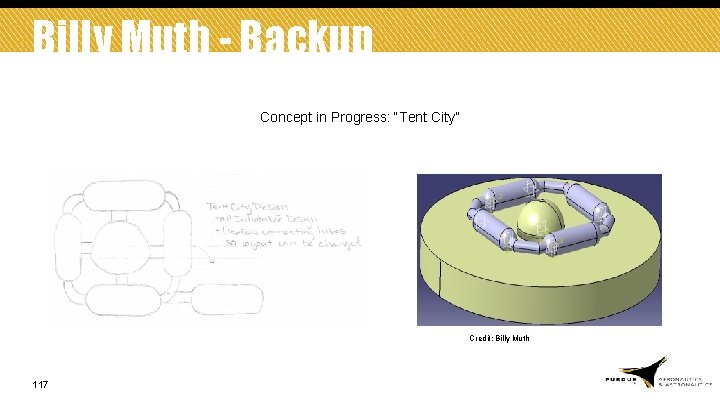
Billy Muth - Backup Concept in Progress: “Tent City” Credit: Billy Muth 117
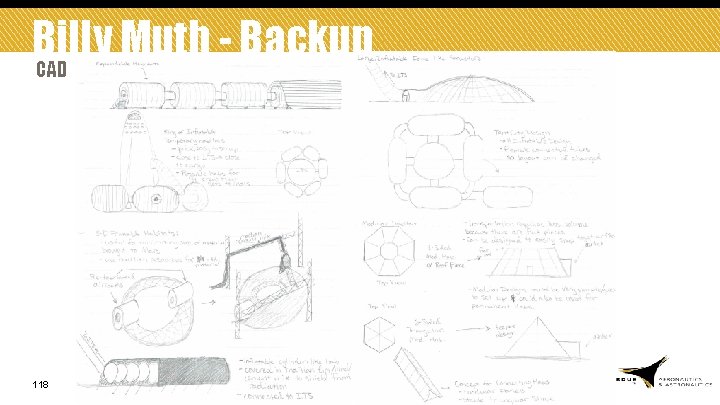
Billy Muth - Backup CAD 118
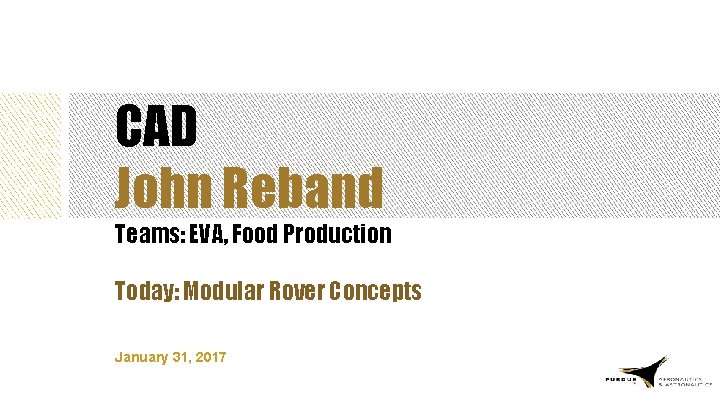
CAD John Reband Teams: EVA, Food Production Today: Modular Rover Concepts January 31, 2017
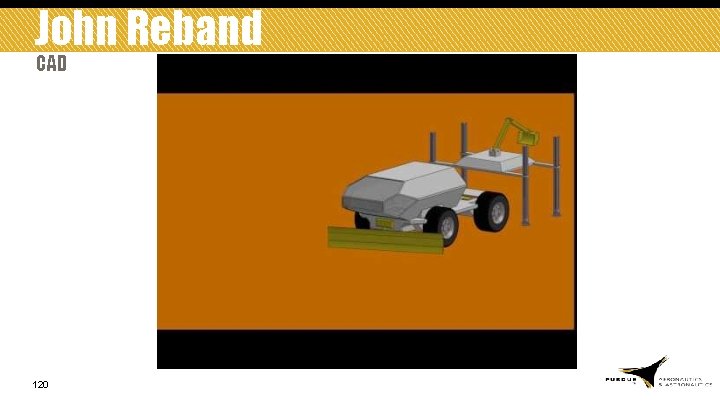
John Reband CAD 120
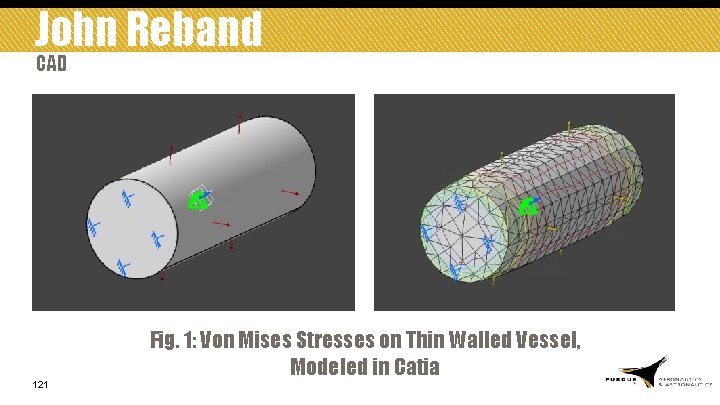
John Reband CAD 121 Fig. 1: Von Mises Stresses on Thin Walled Vessel, Modeled in Catia
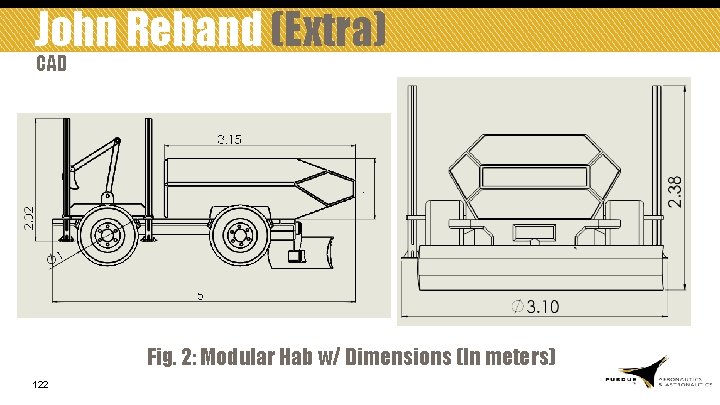
John Reband (Extra) CAD Fig. 2: Modular Hab w/ Dimensions (In meters) 122
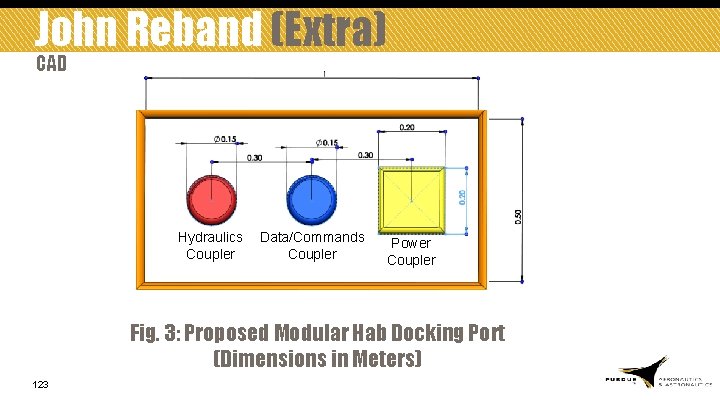
John Reband (Extra) CAD Hydraulics Coupler Data/Commands Coupler Power Coupler Fig. 3: Proposed Modular Hab Docking Port (Dimensions in Meters) 123
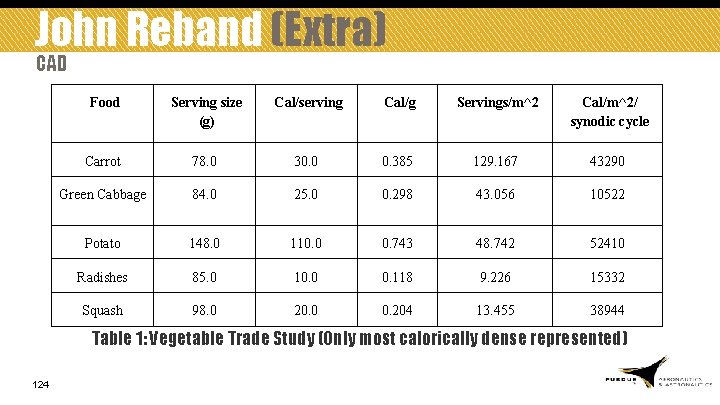
John Reband (Extra) CAD Food Serving size (g) Cal/serving Cal/g Servings/m^2 Cal/m^2/ synodic cycle Carrot 78. 0 30. 0 0. 385 129. 167 43290 Green Cabbage 84. 0 25. 0 0. 298 43. 056 10522 Potato 148. 0 110. 0 0. 743 48. 742 52410 Radishes 85. 0 10. 0 0. 118 9. 226 15332 Squash 98. 0 20. 0 0. 204 13. 455 38944 Table 1: Vegetable Trade Study (Only most calorically dense represented) 124
![John Reband Extra CAD References 1 Vegetable Nutrition Facts fda gov Available http John Reband (Extra) CAD References [1] “Vegetable Nutrition Facts, ” fda. gov Available: http:](https://slidetodoc.com/presentation_image_h/184fd3da0ebff5691a469b53cfd00971/image-125.jpg)
John Reband (Extra) CAD References [1] “Vegetable Nutrition Facts, ” fda. gov Available: http: //www. fda. gov/downloads/Food/Ingredients. Packaging. Labeling/Labeling. N utrition/UCM 169238. pdf. [2] “Planting, Growing, and Harvesting Asparagus, ” almanac. com Available: http: //www. almanac. com/plant/. [3] “Growing Vegetable Gardens, ” Growing Vegetable Gardens Available: Vegetable & Herb Quantity Guidelines. 125
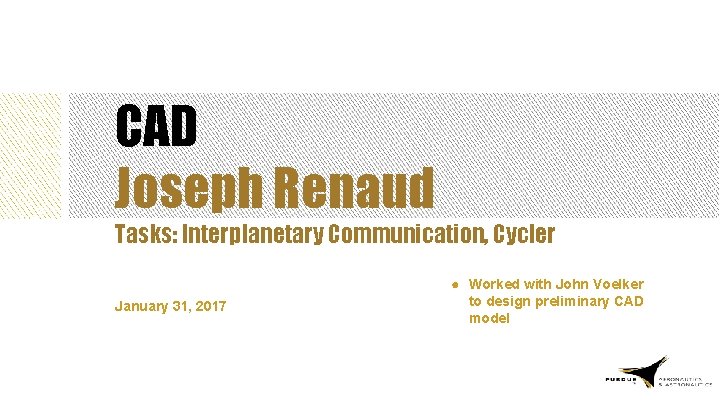
CAD Joseph Renaud Tasks: Interplanetary Communication, Cycler January 31, 2017 ● Worked with John Voelker to design preliminary CAD model
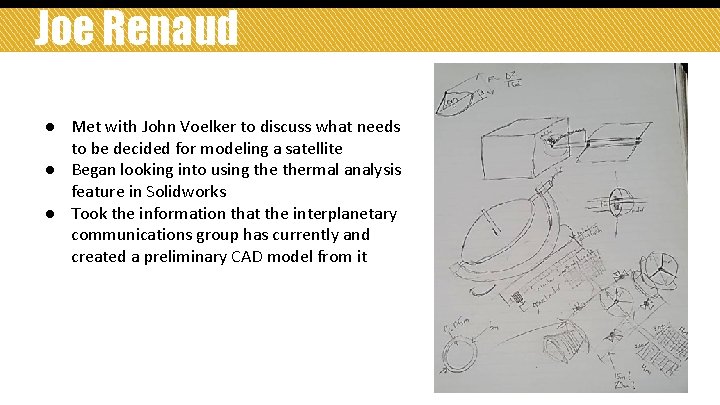
Joe Renaud ● Met with John Voelker to discuss what needs to be decided for modeling a satellite ● Began looking into using thermal analysis feature in Solidworks ● Took the information that the interplanetary communications group has currently and created a preliminary CAD model from it
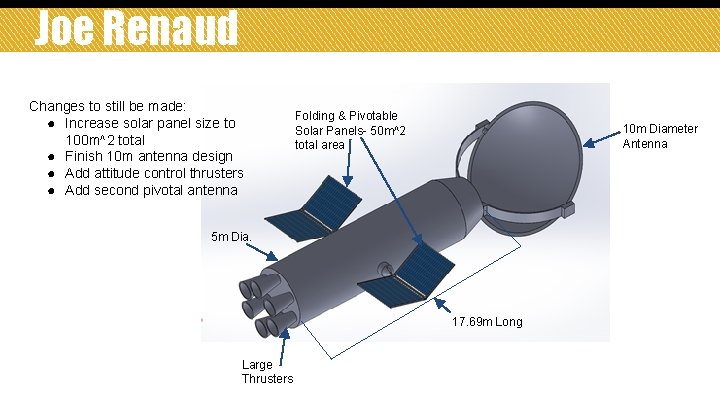
Joe Renaud Changes to still be made: ● Increase solar panel size to 100 m^2 total ● Finish 10 m antenna design ● Add attitude control thrusters ● Add second pivotal antenna Folding & Pivotable Solar Panels- 50 m^2 total area 10 m Diameter Antenna 5 m Dia. 17. 69 m Long Large Thrusters
Gambar trigonometri
Aae 508 purdue
Aae 320
Aae 520
Aae 520
Aae 520
Aae design
Aae 352
Aae 320 uw madison
Aae 320
Aae 251
One purdue
Aae glass tutorial
Aae 421
Purdue aae 590
Aae 421
Manifest destiny def
Craft of scientific presentations
Introduction to mental health awareness presentation
Worst presentation ever powerpoint
Advantages of multimedia presentation
Lecture presentations for campbell biology
Corel presentations ventajas
Developing oral and online presentation
Verbal support in presentations
How to make a tok presentation
World's worst presentation
Anna ritchie allan
Setting up ria
Boardworks ltd
Pyramid principle presentations
Internet presentations
The most dangerous game powerpoint presentations
Note cards for presentations
The end pictures for presentations
Useful phrases for presentations
Efficient elements license key
You exec
Research project title
Catchy titles for presentations
Military robots ppt
Customer service presentations
3750 stack
Bad powerpoint presentations examples
Really bad powerpoint
You exec
Roof ppt presentations
Yoursite com
Horse topics for presentations
Types of oral presentations
Example of work immersion in barangay
Is had a noun
Studies weekly online
Kanban weekly meeting
Cleo weekly quiz
Avid interactive notebook
Andy walton weekly world watch
Longrich compensation plan
Bi-weekly call
Andy waltons weekly world watch
Weekly world watch
The new york weekly journal
Weekly homework assignment sheet
First grade weekly newsletter
The new york weekly journal
Weekly definition
Mean median mode data analysis
7 regions of alabama
Mr potato
Weekly assignment sheet
Spp tcr calendar
Kindergarten weekly newsletter
Cern it department
Andy waltons weekly world watch
Weekly battle rhythm
Teamwork in work immersion
Pear deck weekly wonders
Ichimoku cloud review
Ichimoku
Marking tools in sewing
Tom has a weekly allowance of $24 all of which
What is the name
Weekly objectives meaning
Statistics
Medical transportation ri
Floor covering weekly top 50
Comsae format
Prime factorization of 84 using exponents
450 bc rome
450/1100
450 000 000 in scientific notation
Fortus 450mc price
450 check example
Kj 450
Un termo contiene 450 g de cafe a 85
Ece 450
452 en yakın yüzlüğe yuvarlama
Setrvačník koná 450 otáček za minutu
90 nın yüzde 20'si kaçtır
450 ad
Tennessee sales and use tax blanket certificate of resale
Pixi aiming for grade 5 answers
450 scenario
Ley 450
League of legends jeopardy 2020
Setrvačník koná 450 otáček za minutu
450° angle
460 en yakın onluğa yuvarlama
Ece 450
Ece 450
Old english anglo saxon period
Itrs 로드맵
Trapped zeros
Im 450
Old english (450-1150)
Old english (450-1100 ad)
Proste a i b są równoległe półproste pa i pb
Comcst cable
Artificial intelligence
A & d window cleaning performed $450 of services
Konversikan hasil pengukuran berikut
Asc 450 decision tree
Harry regan and kelan share £450
Norma 450
Besar setiap sudut segilima
Julia tiene 450 soles y decide ahorrar el 30
Jlg 450 hidraulična zglobna platforma
Jorge grcia
Musical representation of specific poetic images