PSCI 1414 GENERAL ASTRONOMY THE SUN PART 3
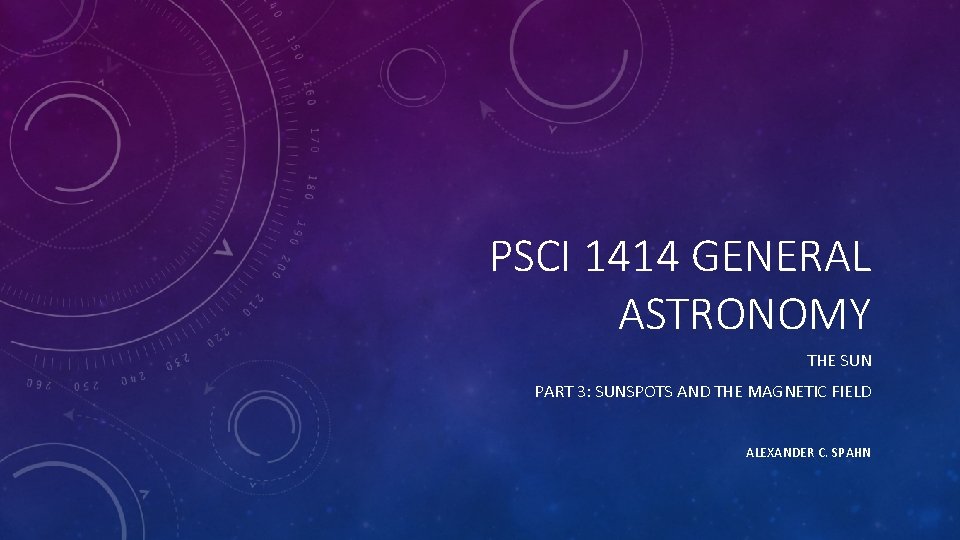
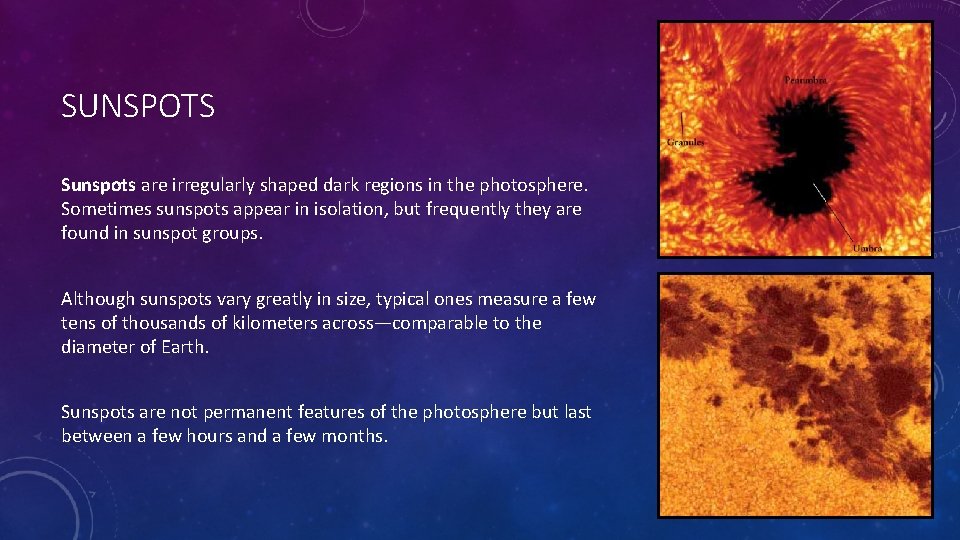
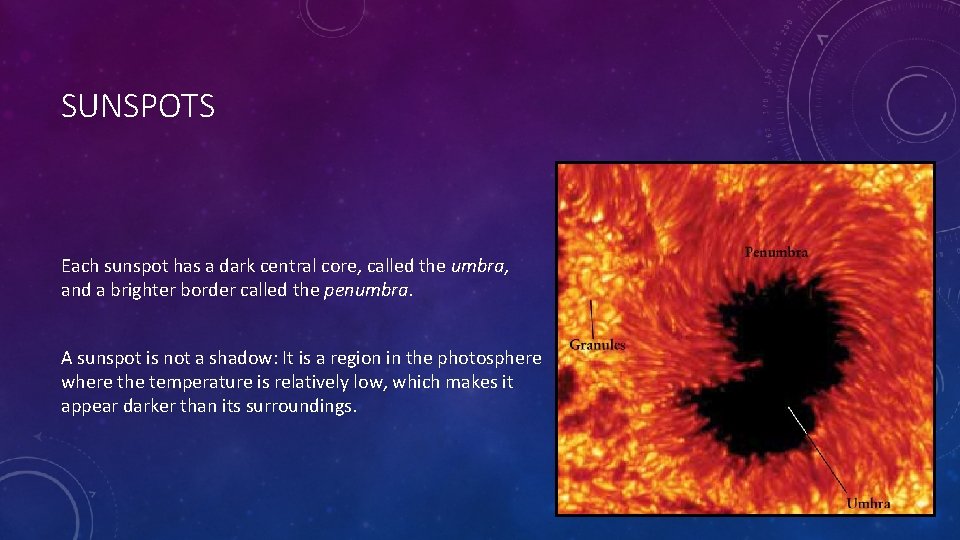
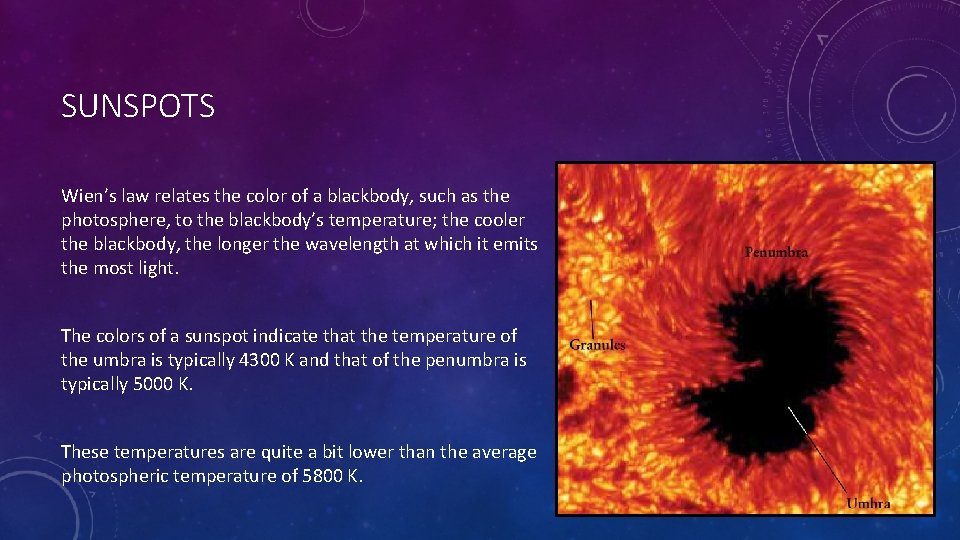
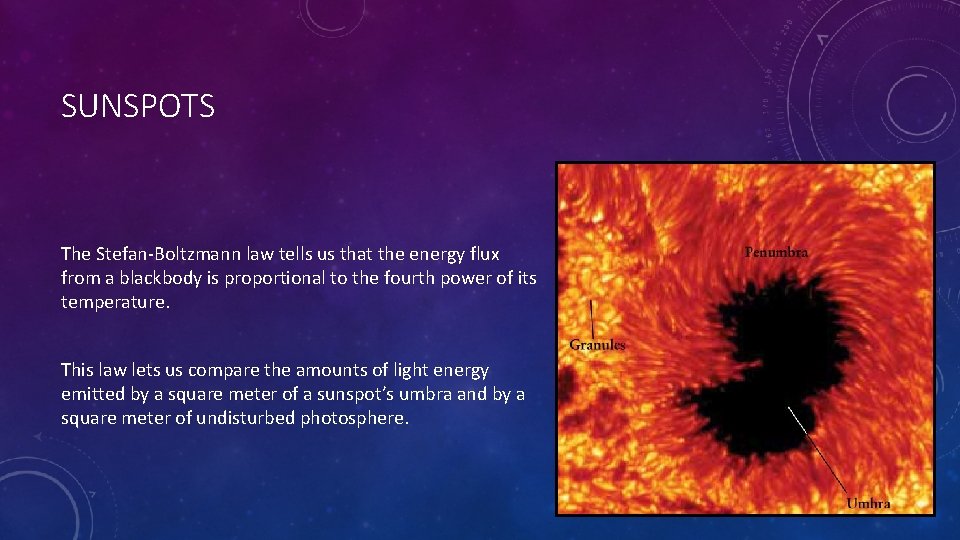
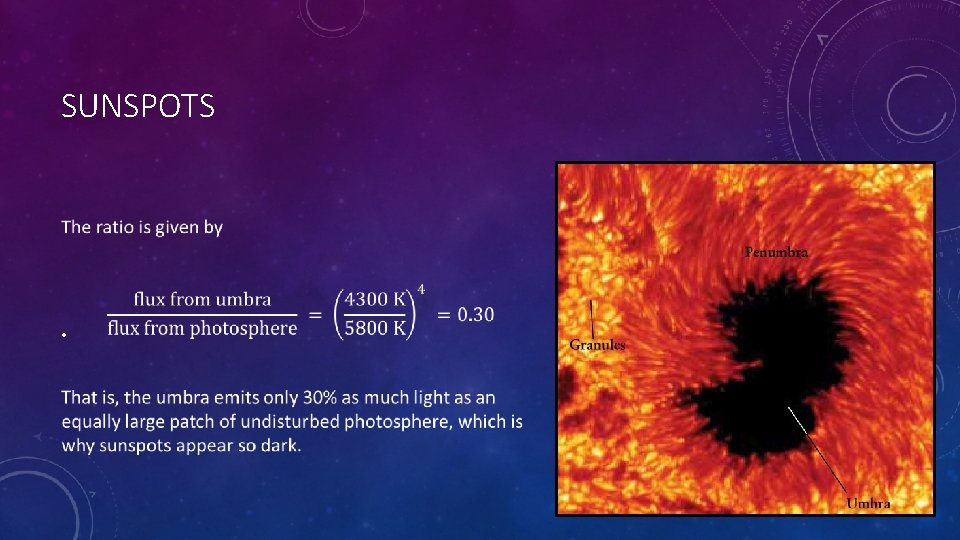
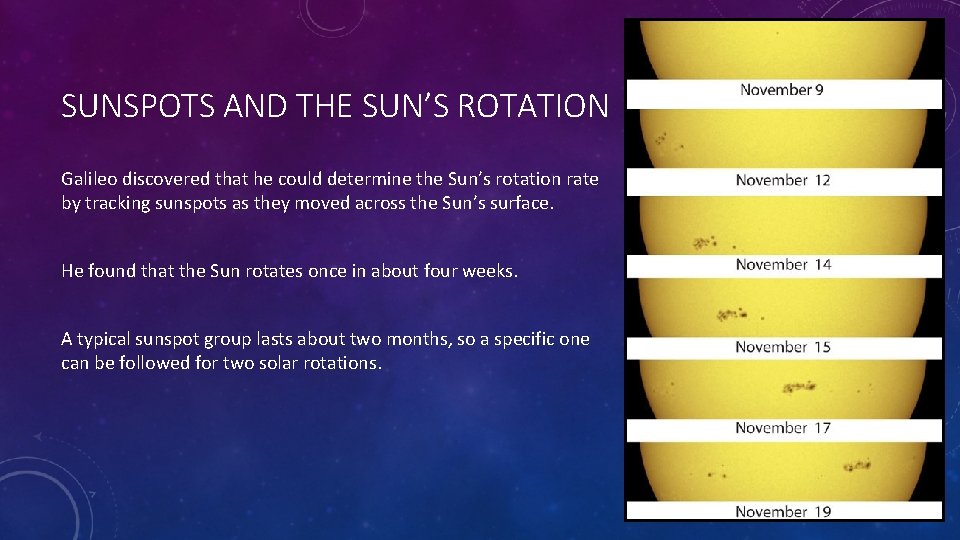
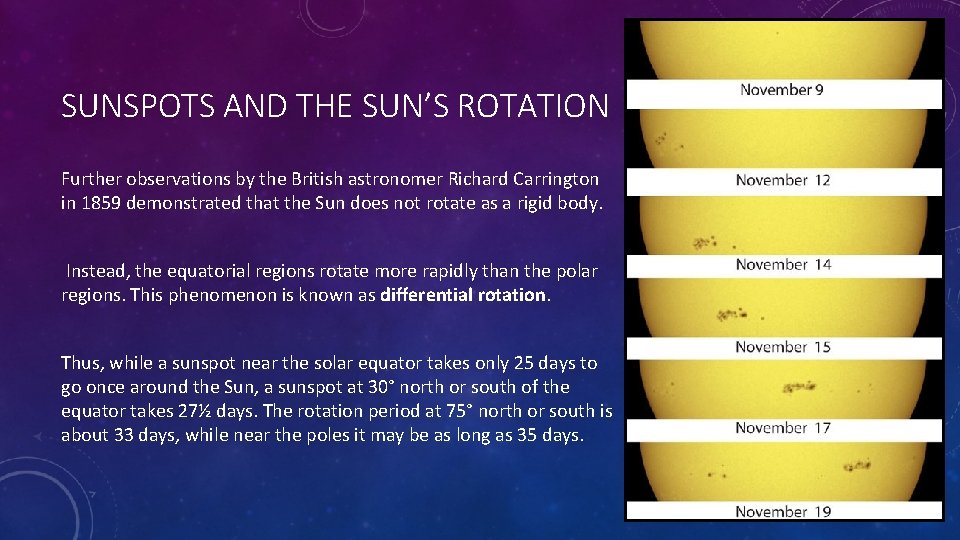
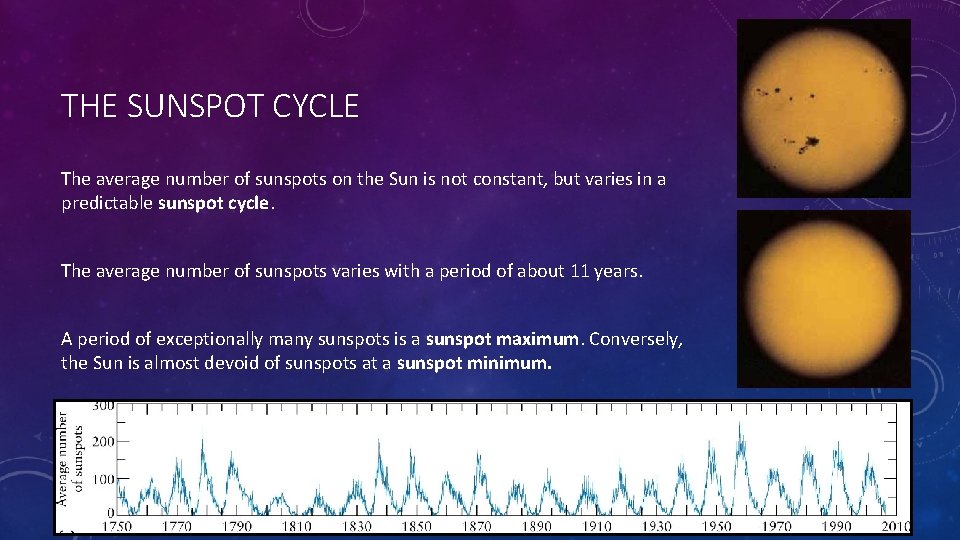
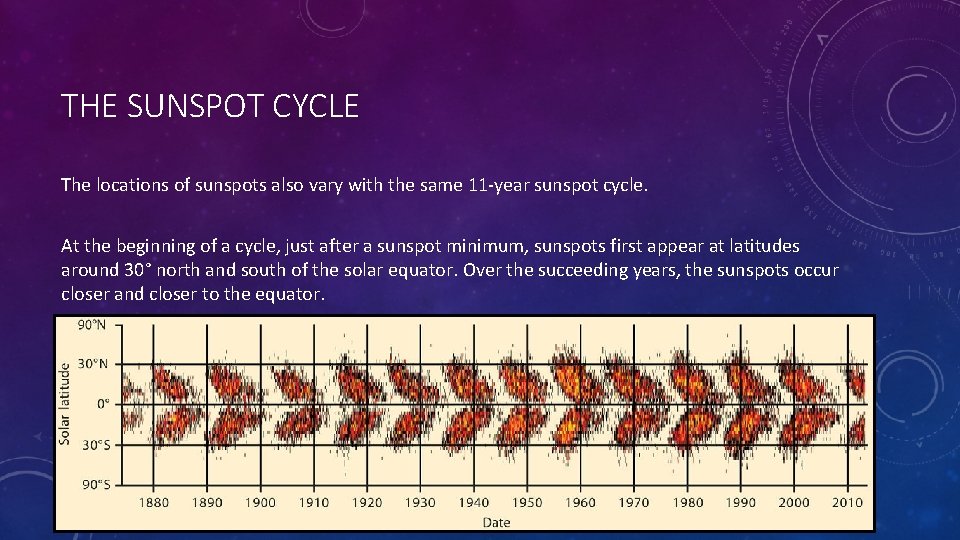
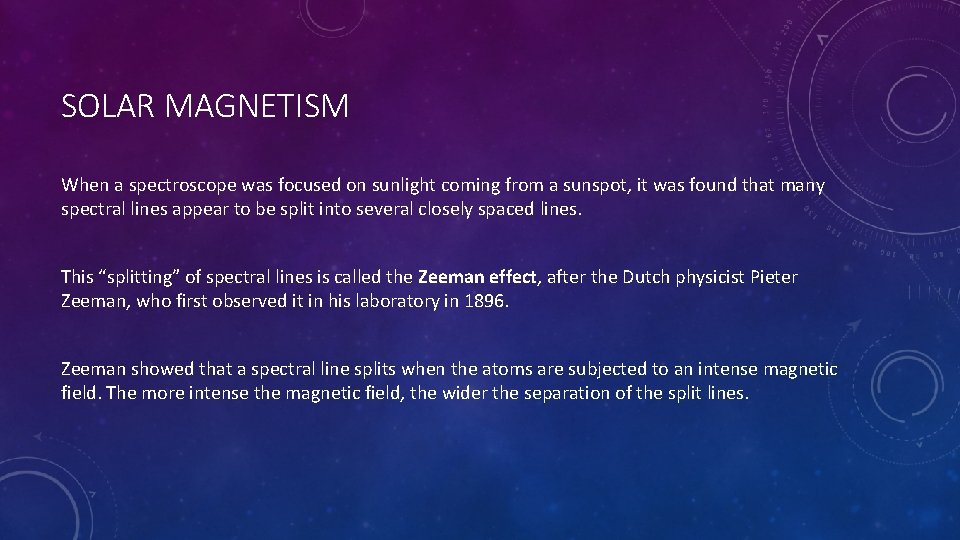
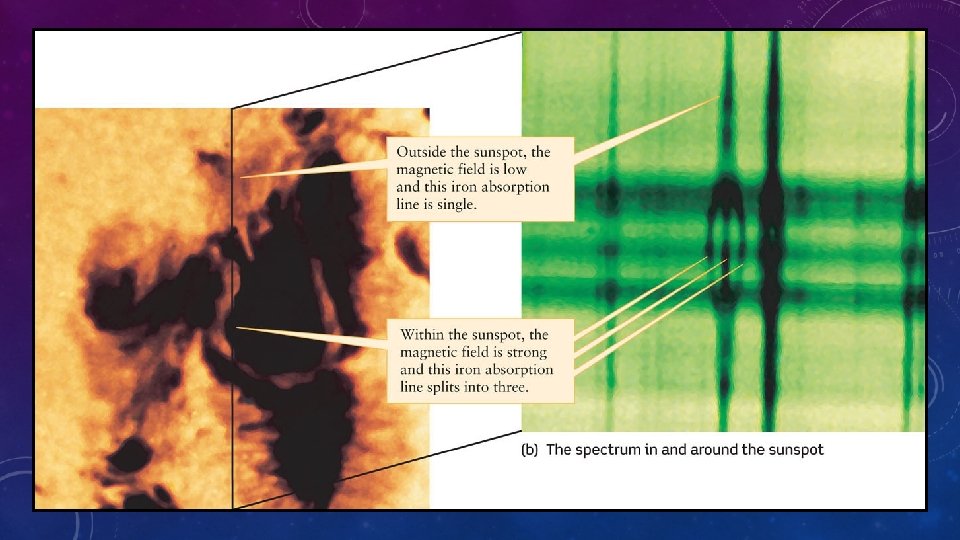
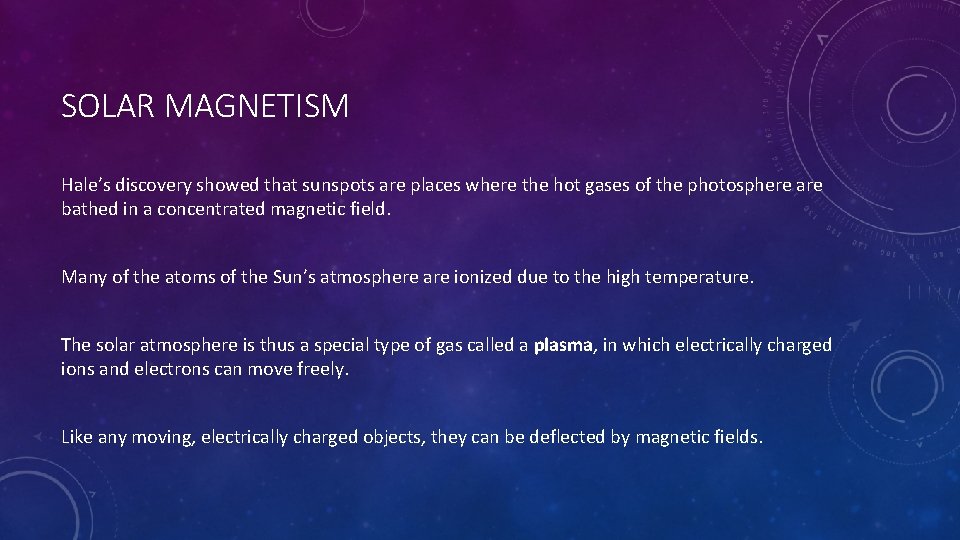
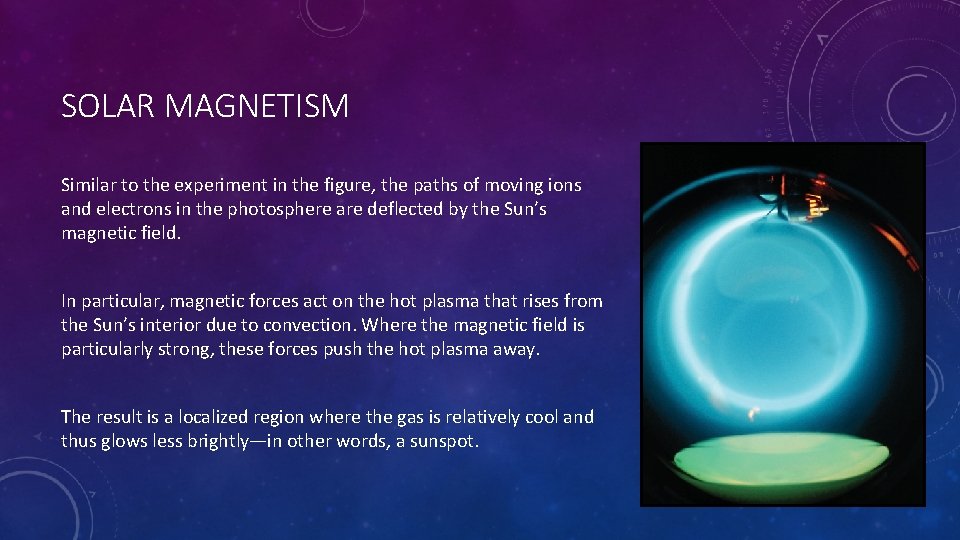
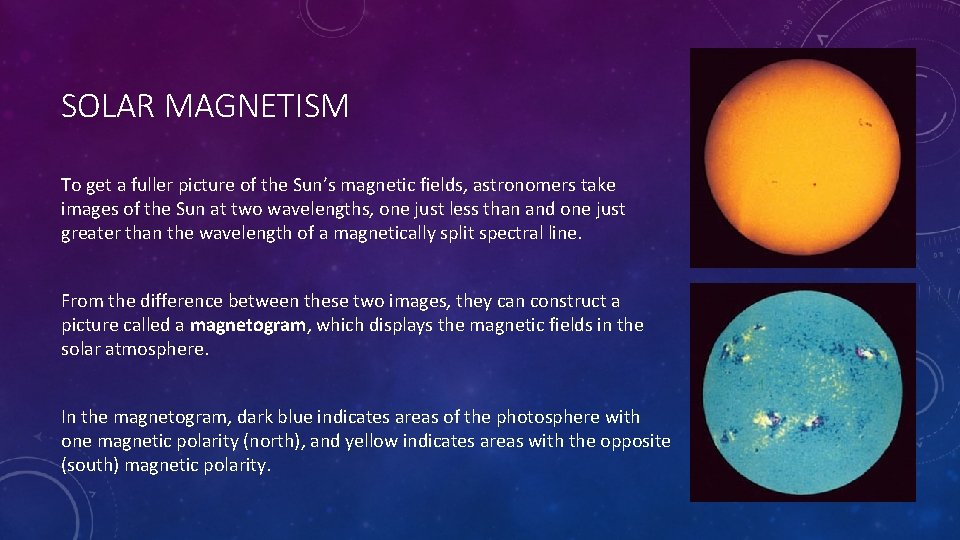
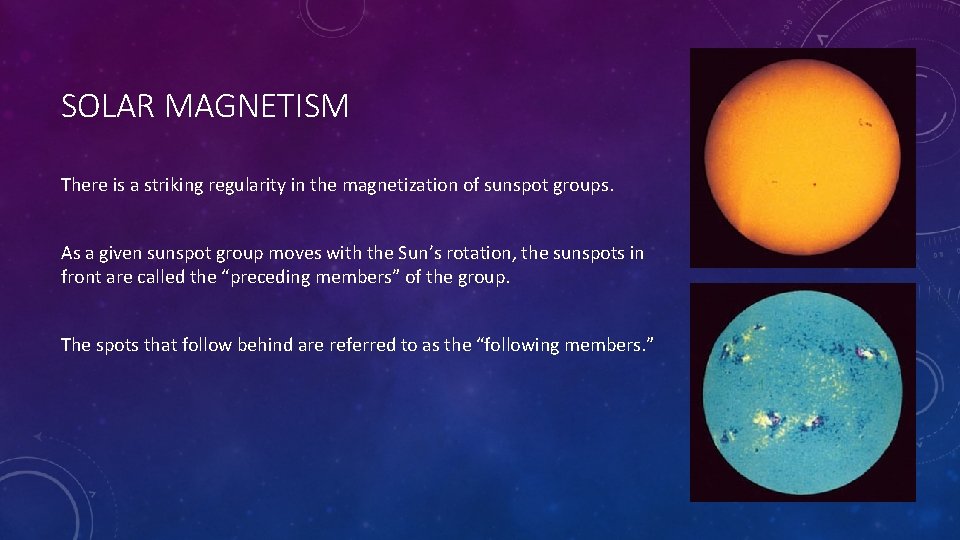
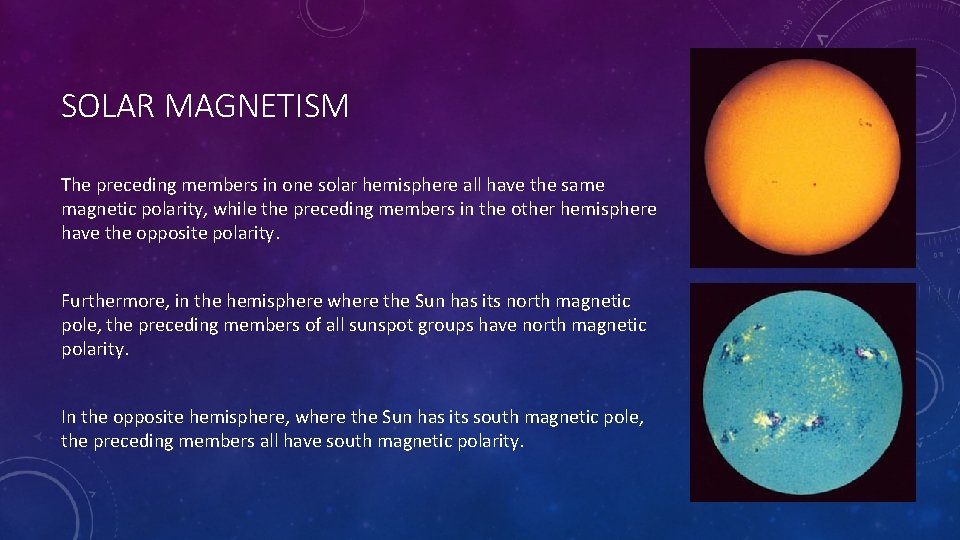
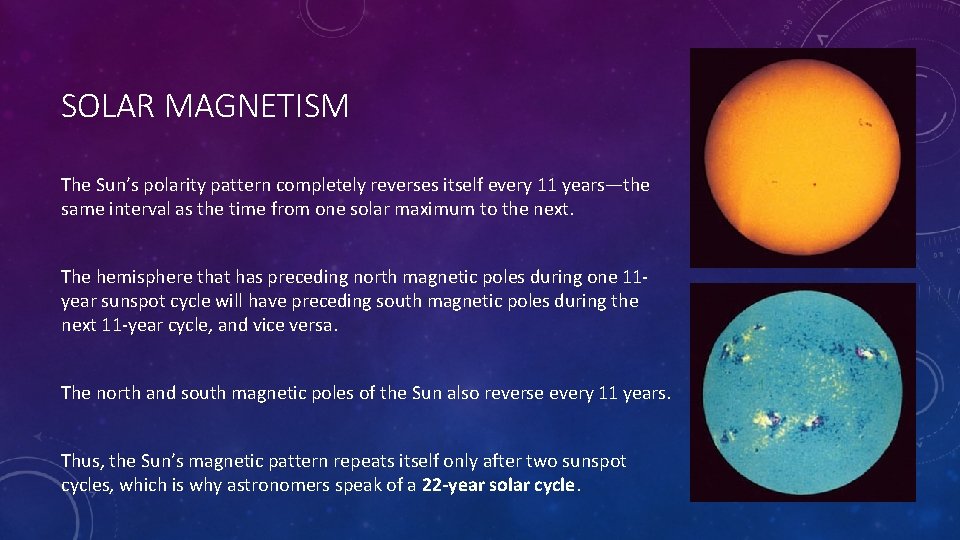
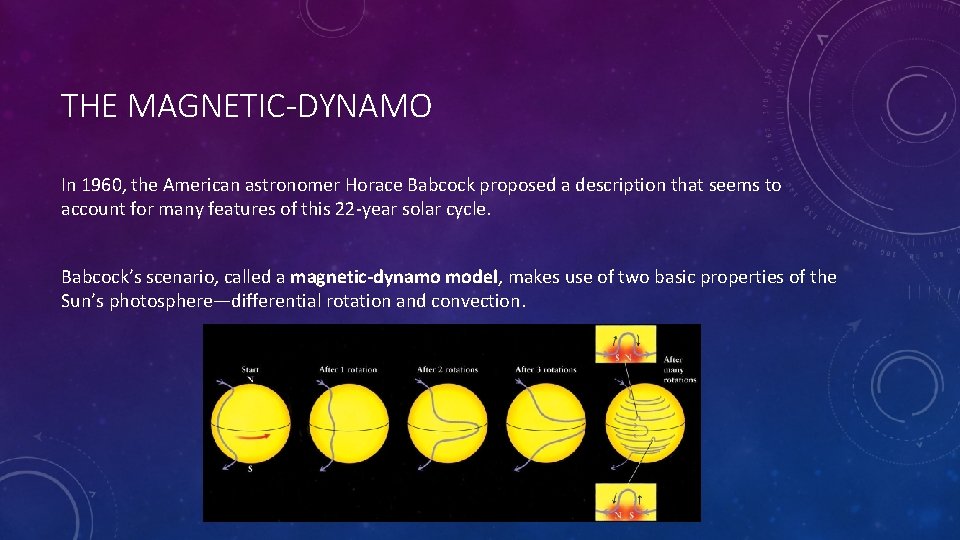
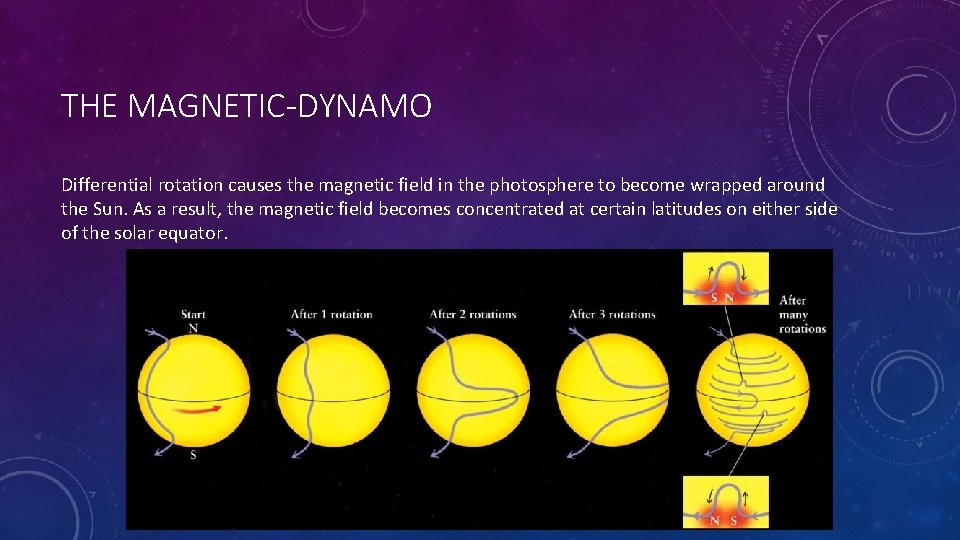
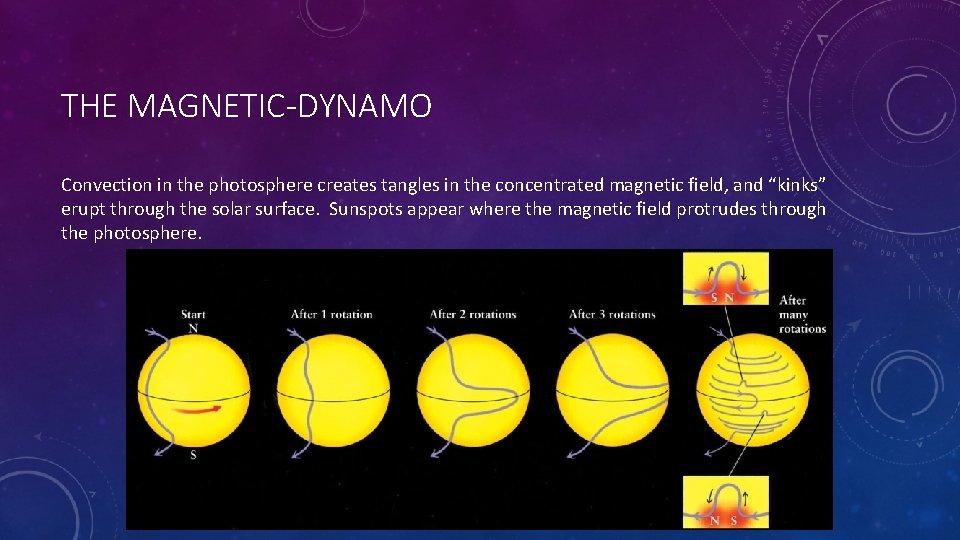
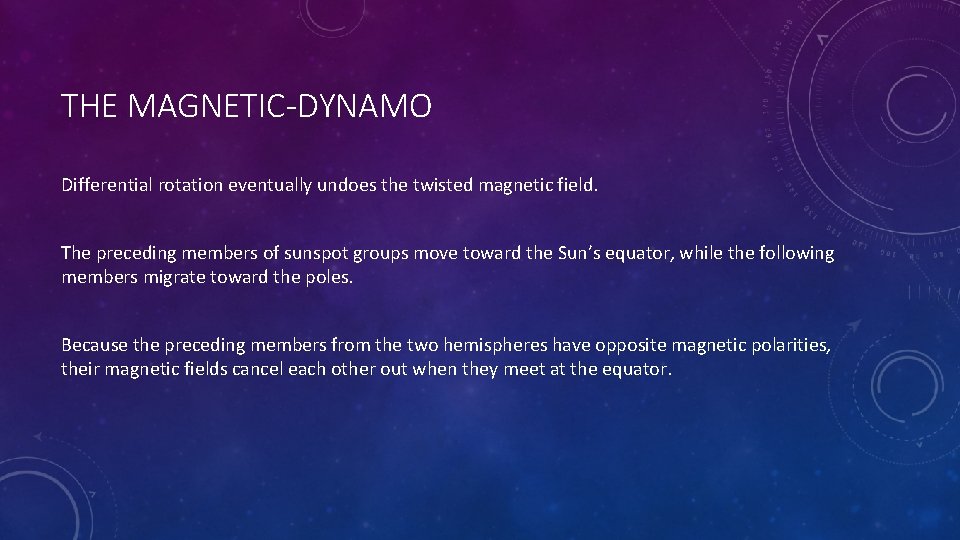
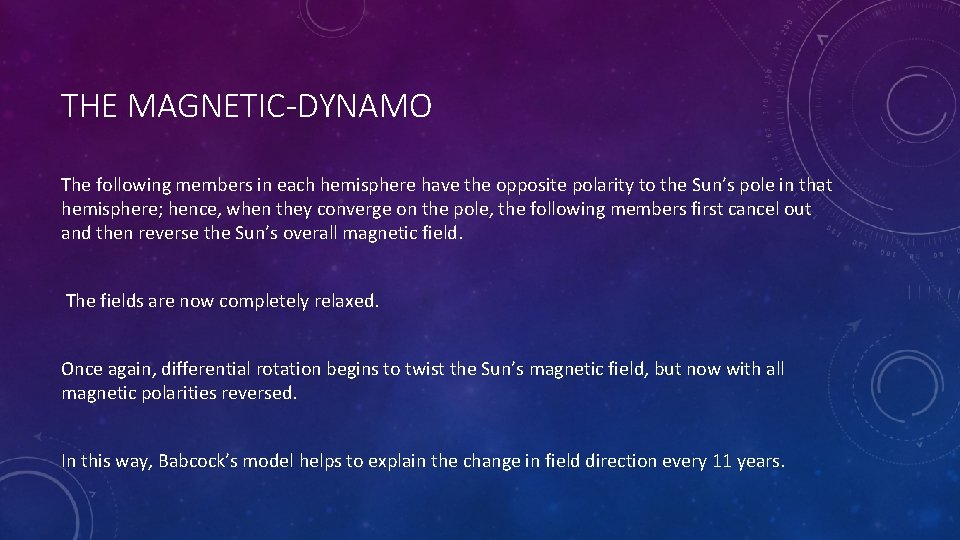
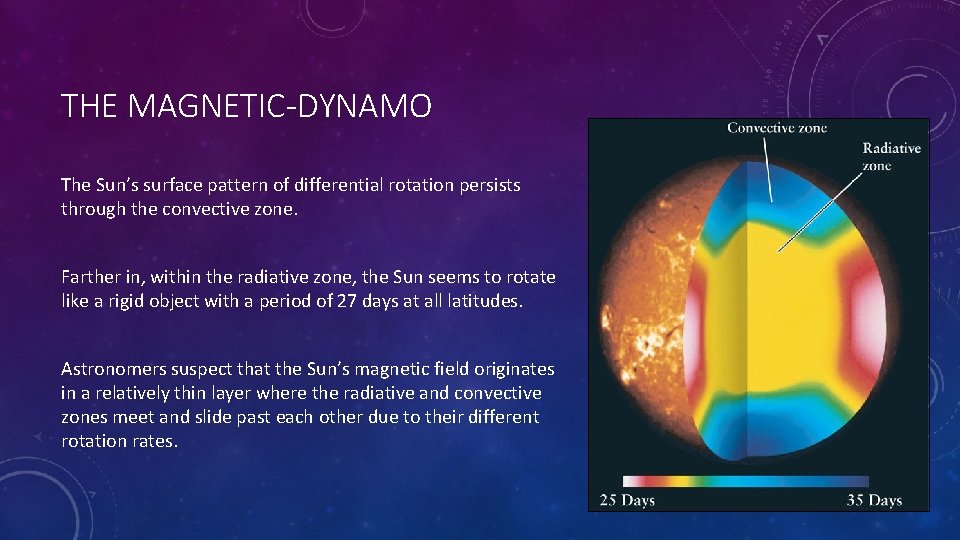
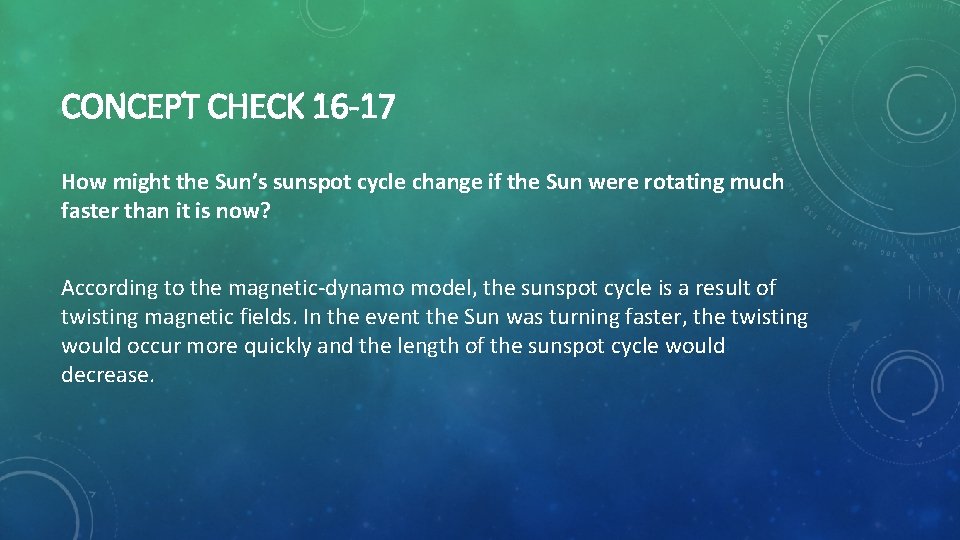
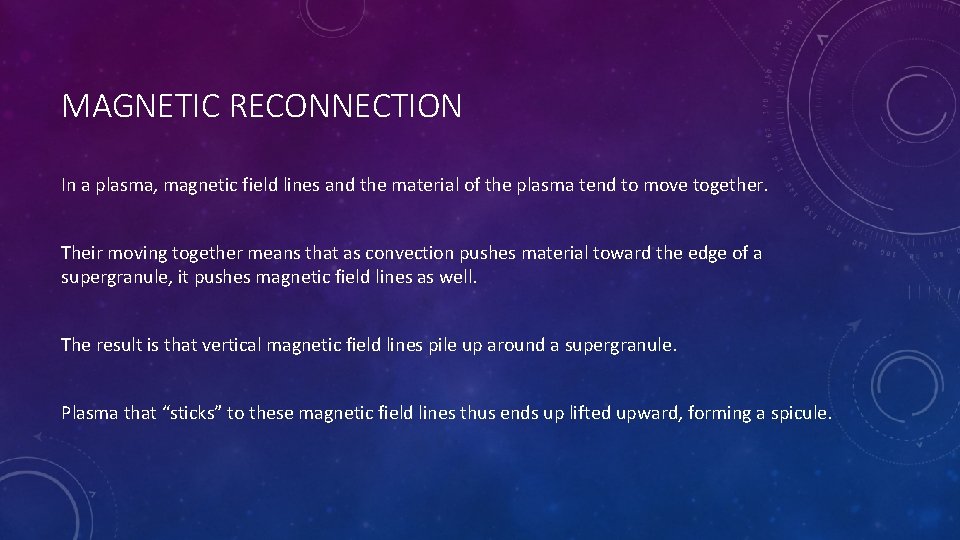
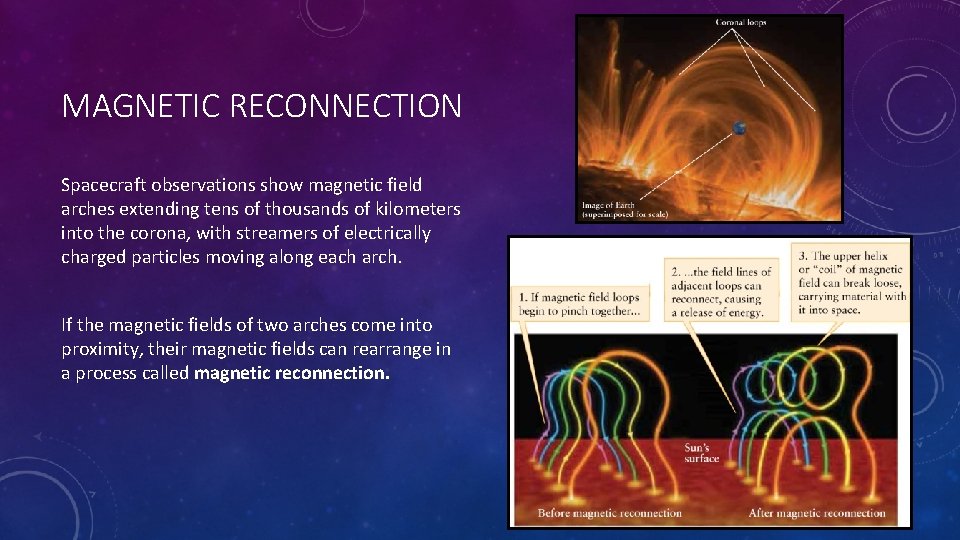
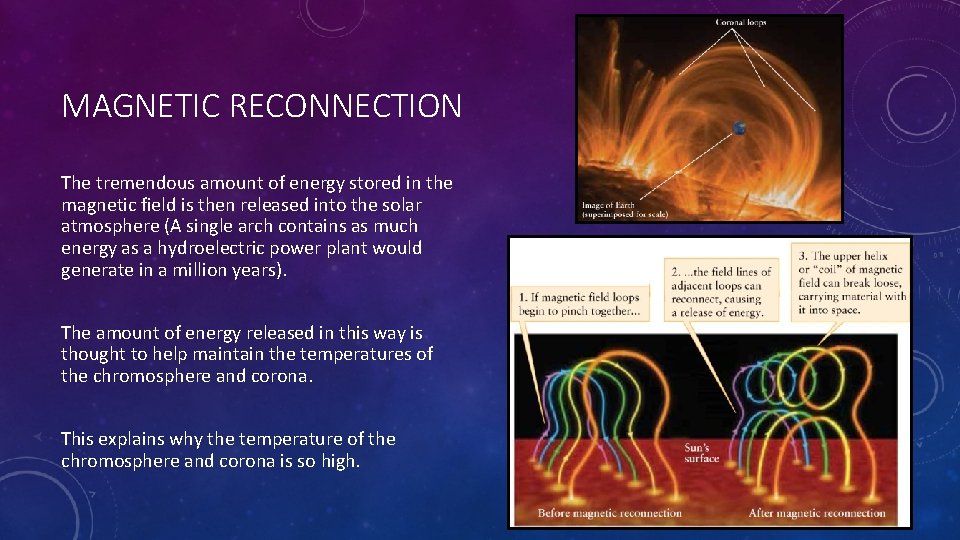
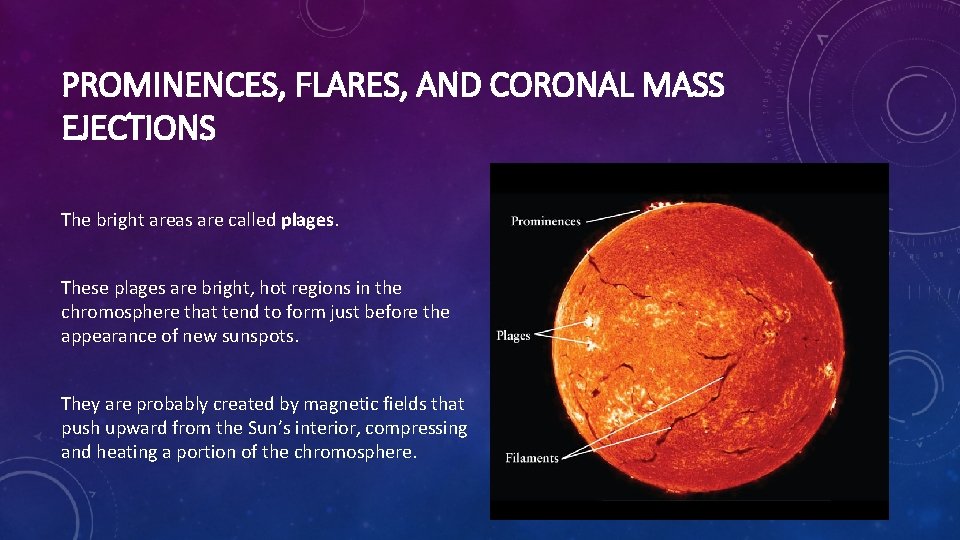
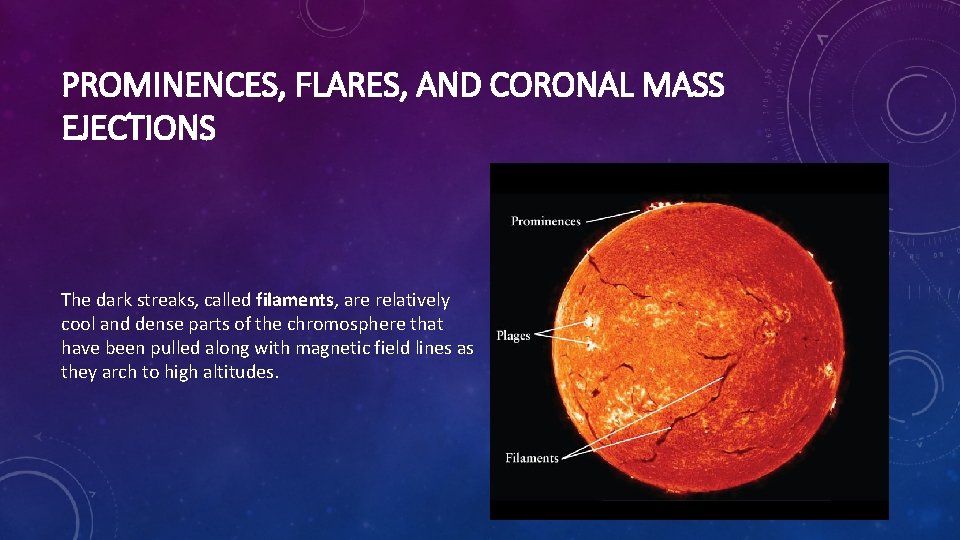
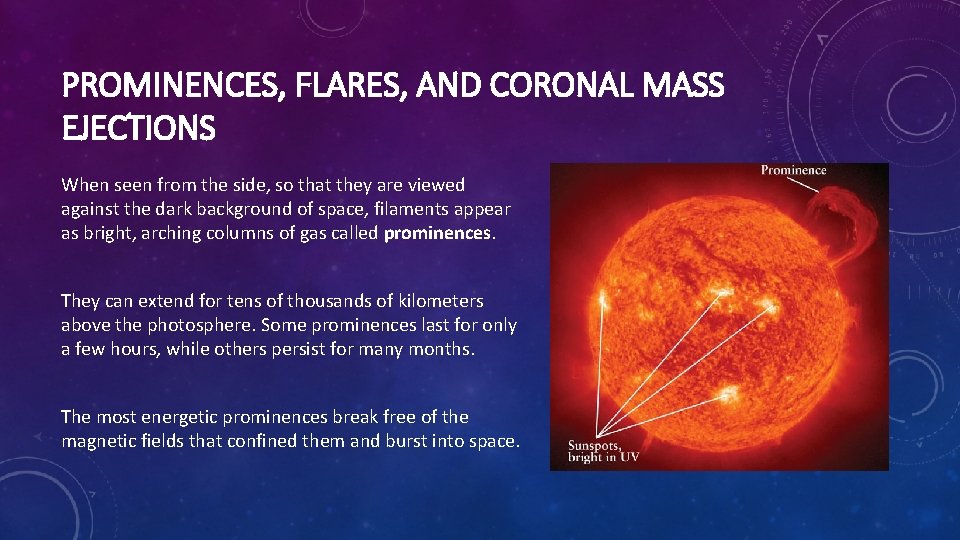
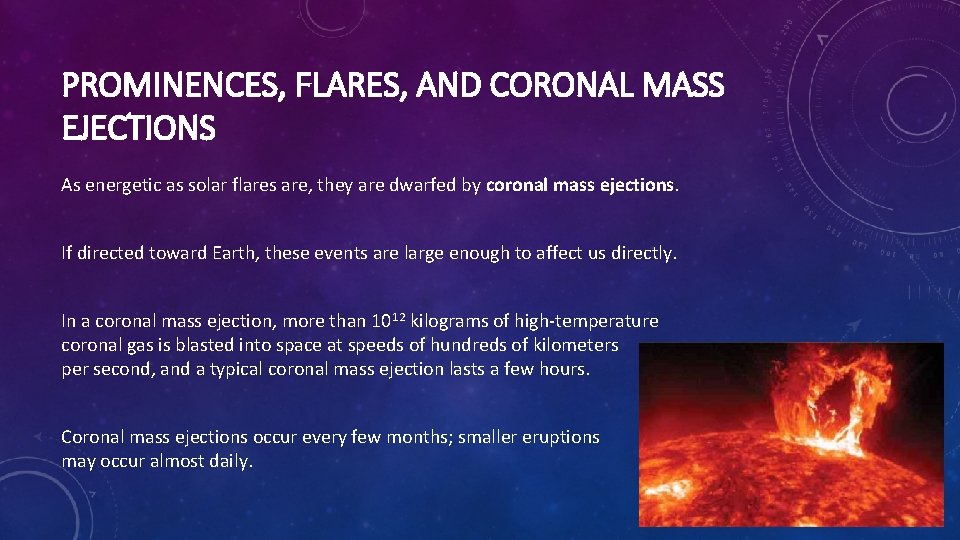
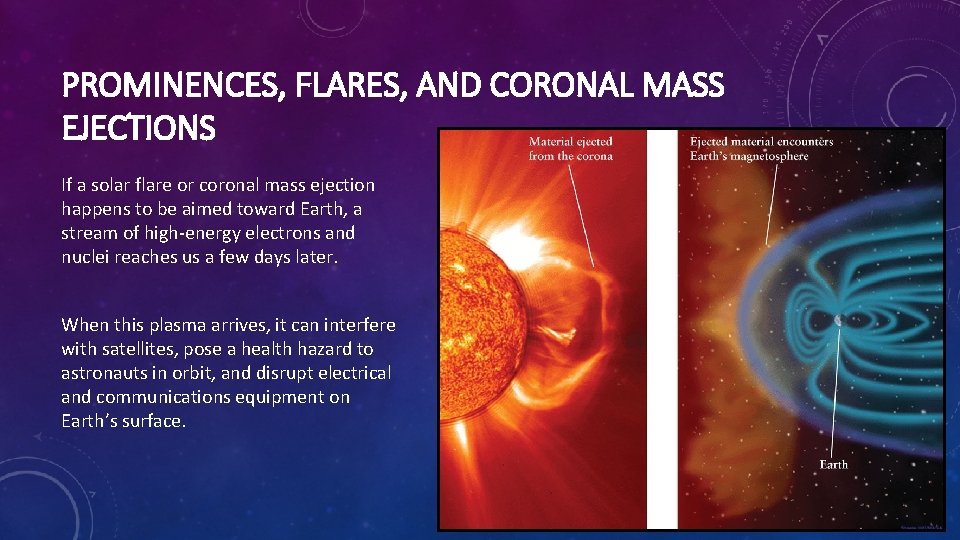
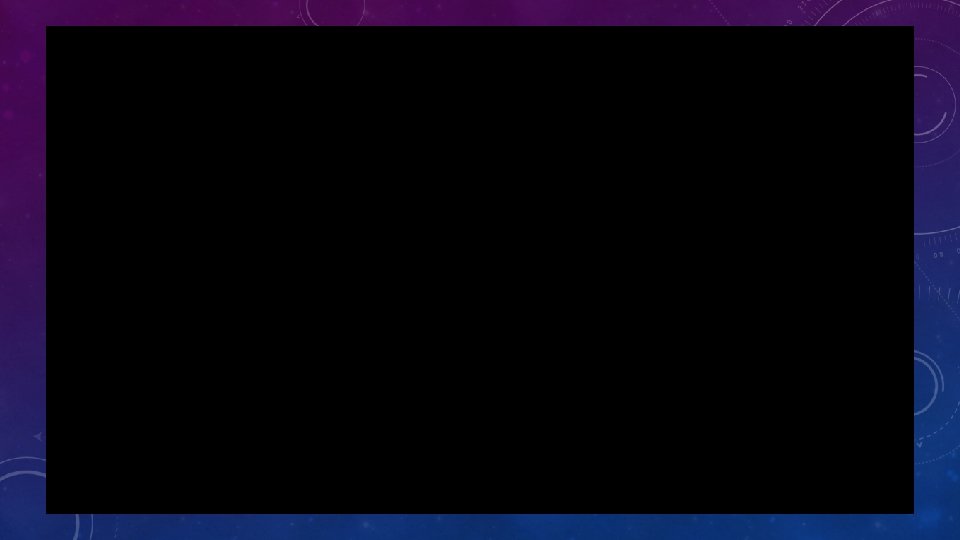
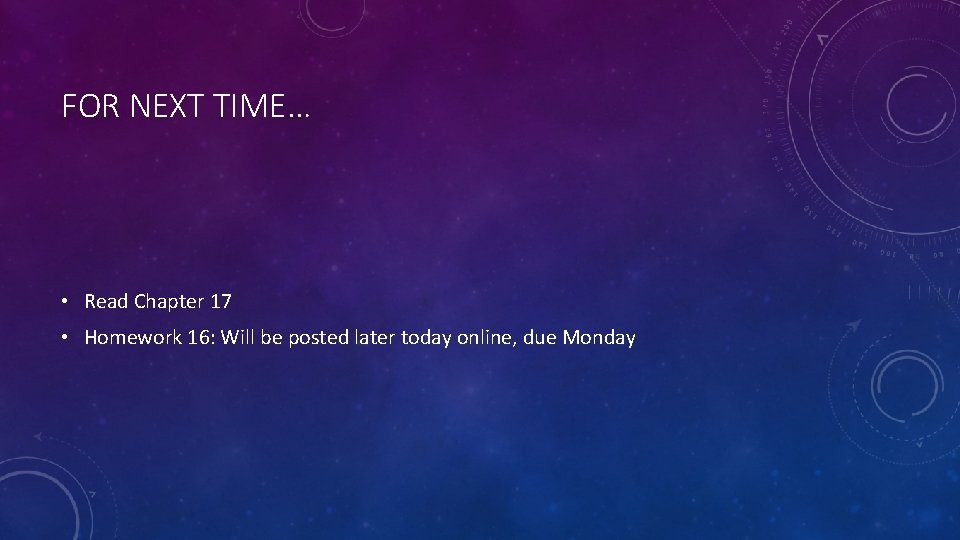
- Slides: 35
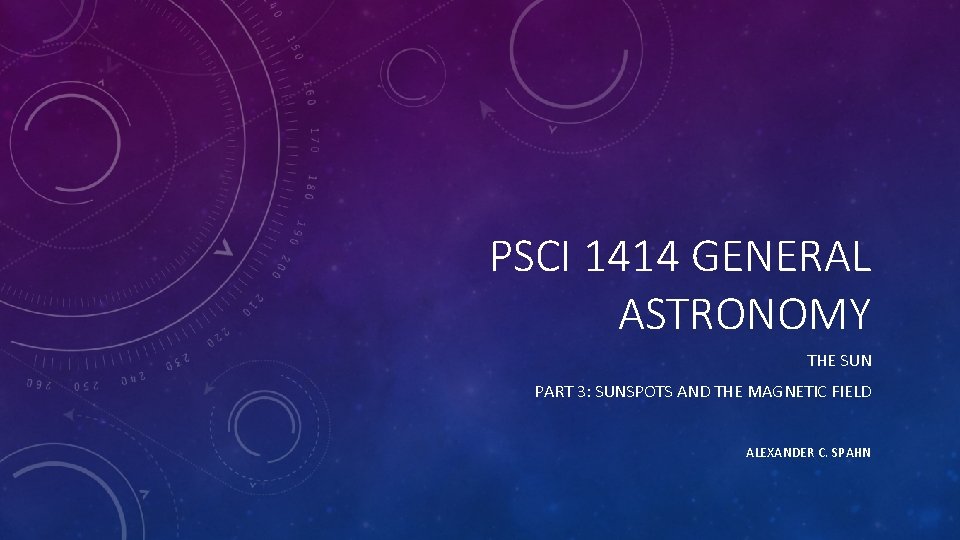
PSCI 1414 GENERAL ASTRONOMY THE SUN PART 3: SUNSPOTS AND THE MAGNETIC FIELD ALEXANDER C. SPAHN
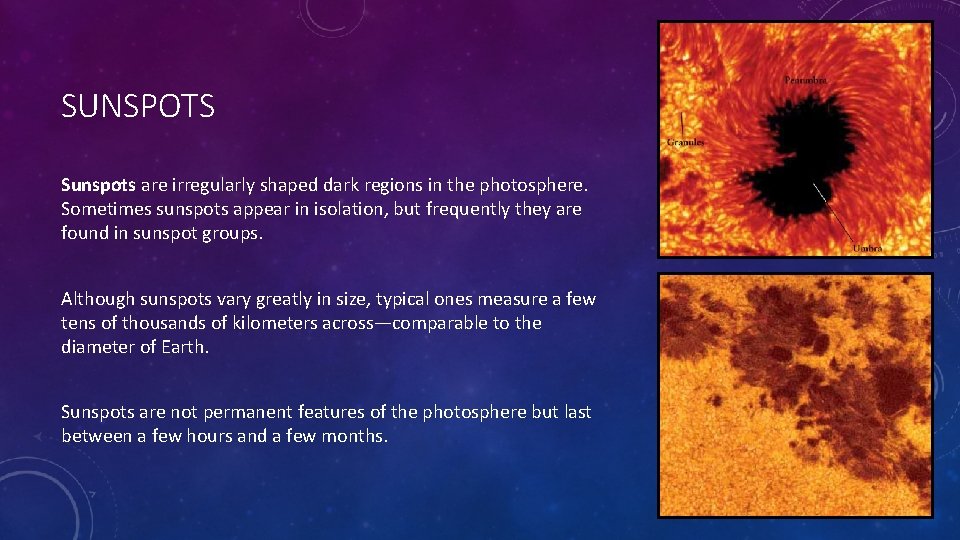
SUNSPOTS Sunspots are irregularly shaped dark regions in the photosphere. Sometimes sunspots appear in isolation, but frequently they are found in sunspot groups. Although sunspots vary greatly in size, typical ones measure a few tens of thousands of kilometers across—comparable to the diameter of Earth. Sunspots are not permanent features of the photosphere but last between a few hours and a few months.
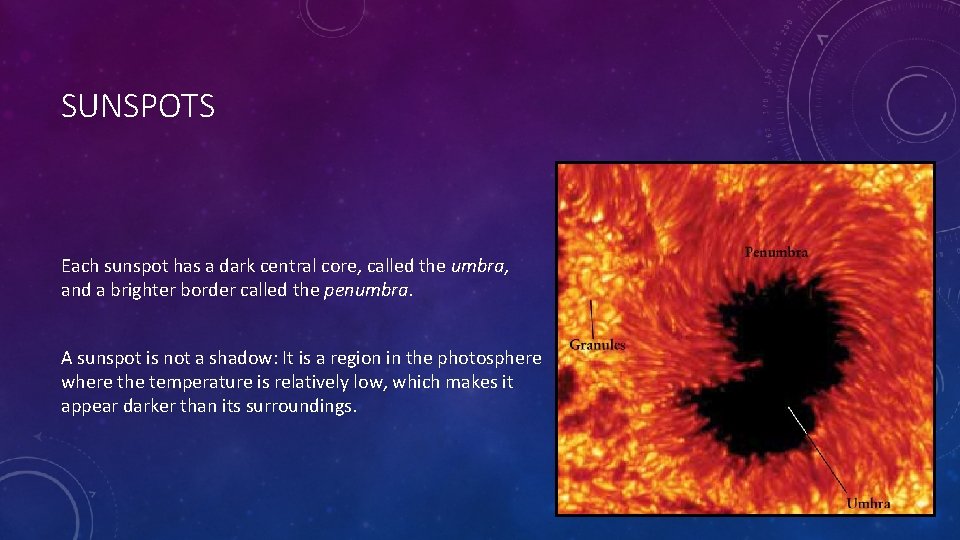
SUNSPOTS Each sunspot has a dark central core, called the umbra, and a brighter border called the penumbra. A sunspot is not a shadow: It is a region in the photosphere where the temperature is relatively low, which makes it appear darker than its surroundings.
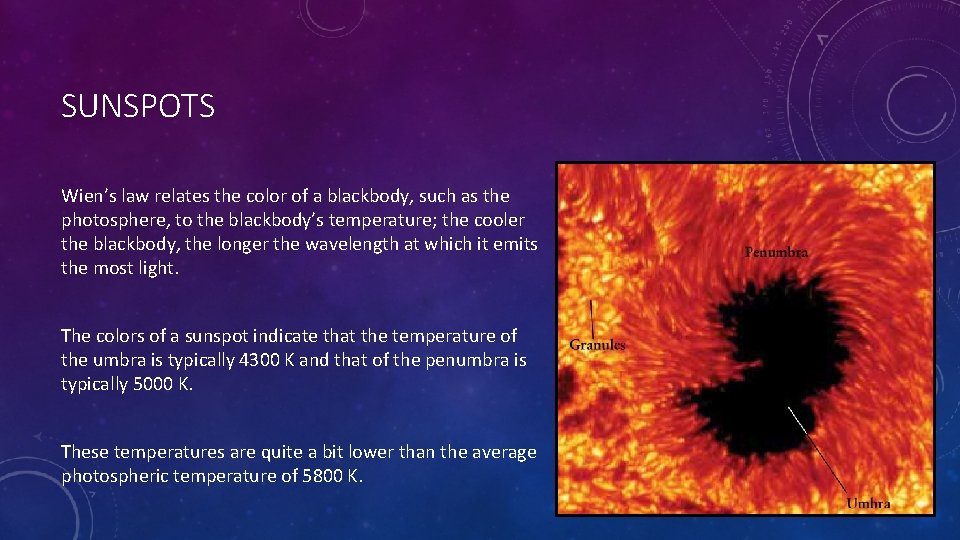
SUNSPOTS Wien’s law relates the color of a blackbody, such as the photosphere, to the blackbody’s temperature; the cooler the blackbody, the longer the wavelength at which it emits the most light. The colors of a sunspot indicate that the temperature of the umbra is typically 4300 K and that of the penumbra is typically 5000 K. These temperatures are quite a bit lower than the average photospheric temperature of 5800 K.
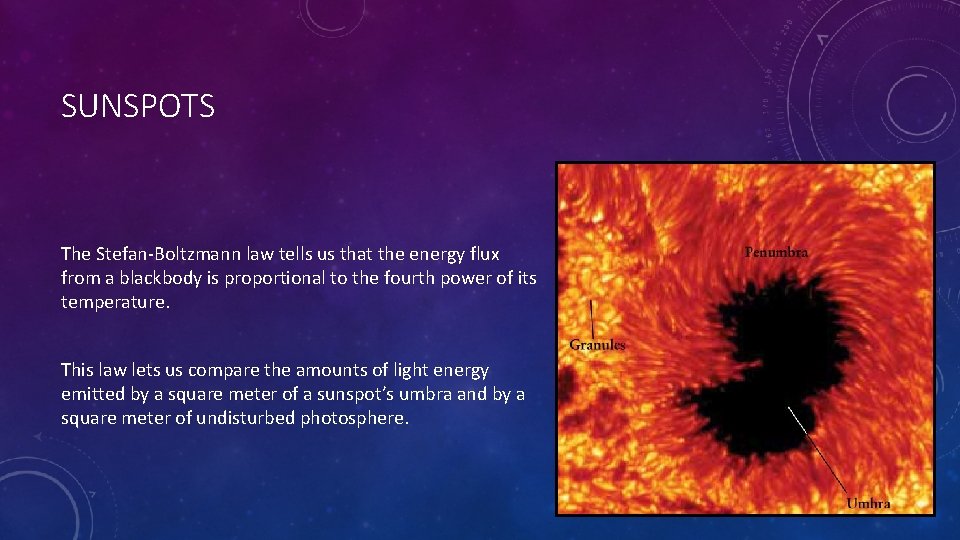
SUNSPOTS The Stefan-Boltzmann law tells us that the energy flux from a blackbody is proportional to the fourth power of its temperature. This law lets us compare the amounts of light energy emitted by a square meter of a sunspot’s umbra and by a square meter of undisturbed photosphere.
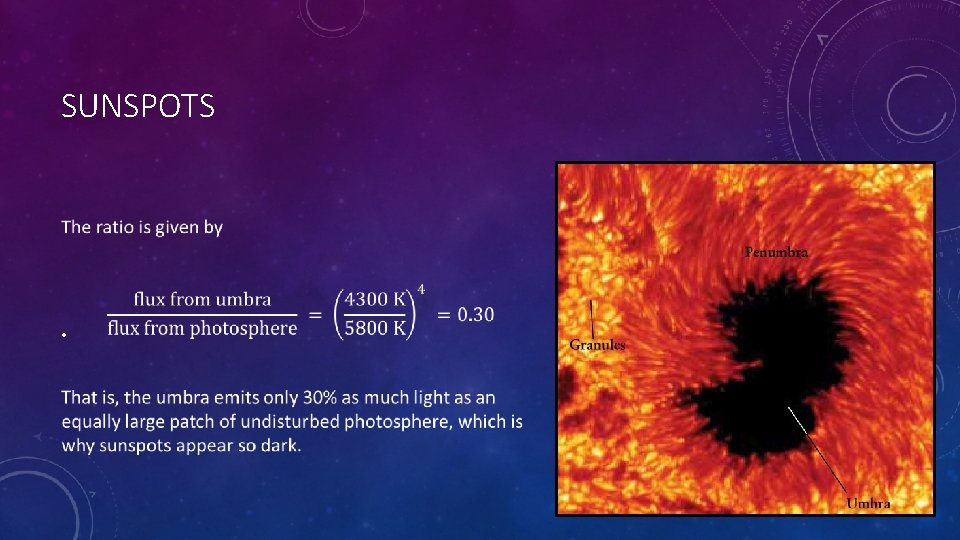
SUNSPOTS •
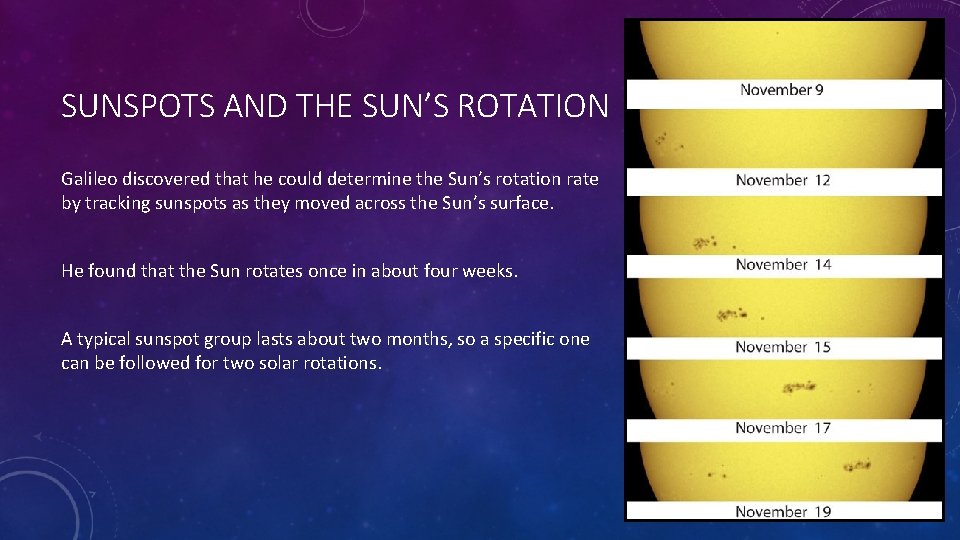
SUNSPOTS AND THE SUN’S ROTATION Galileo discovered that he could determine the Sun’s rotation rate by tracking sunspots as they moved across the Sun’s surface. He found that the Sun rotates once in about four weeks. A typical sunspot group lasts about two months, so a specific one can be followed for two solar rotations.
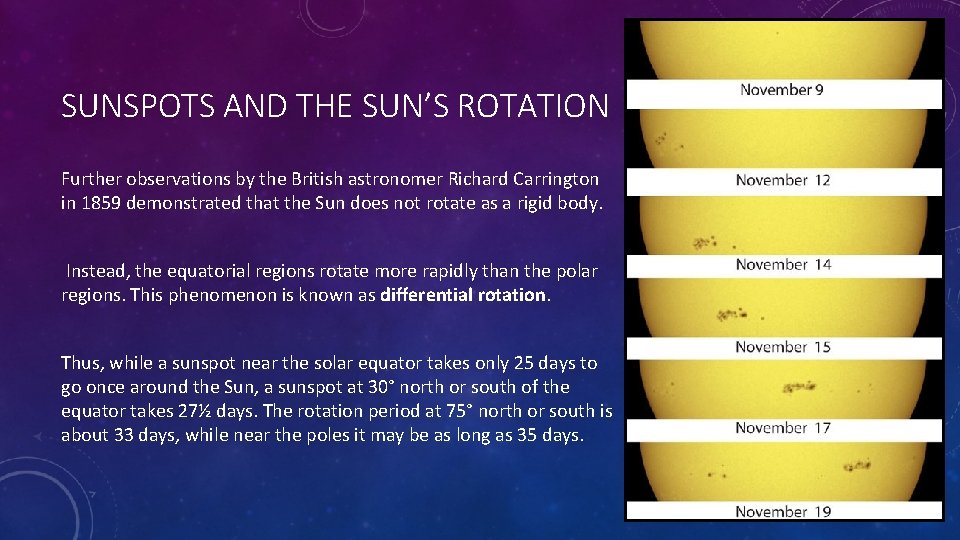
SUNSPOTS AND THE SUN’S ROTATION Further observations by the British astronomer Richard Carrington in 1859 demonstrated that the Sun does not rotate as a rigid body. Instead, the equatorial regions rotate more rapidly than the polar regions. This phenomenon is known as differential rotation. Thus, while a sunspot near the solar equator takes only 25 days to go once around the Sun, a sunspot at 30° north or south of the equator takes 27½ days. The rotation period at 75° north or south is about 33 days, while near the poles it may be as long as 35 days.
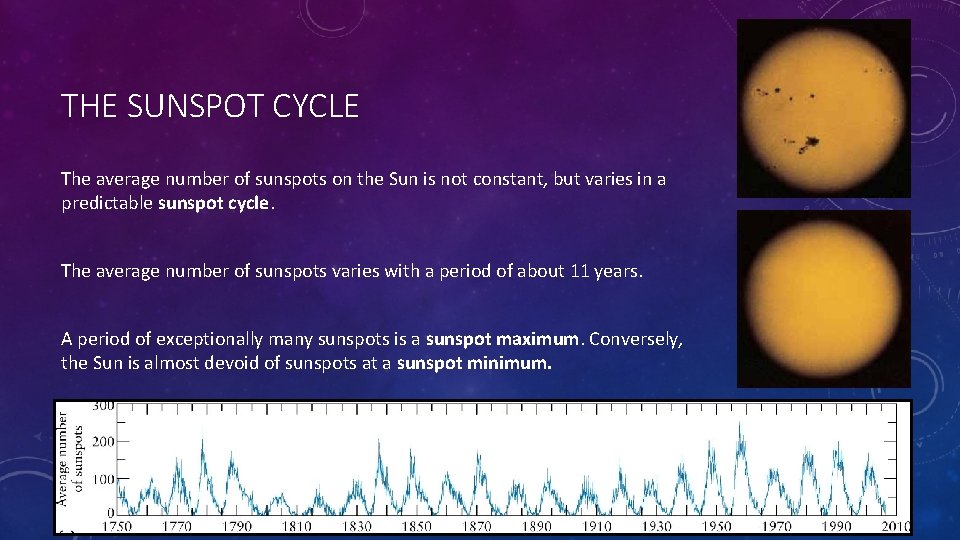
THE SUNSPOT CYCLE The average number of sunspots on the Sun is not constant, but varies in a predictable sunspot cycle. The average number of sunspots varies with a period of about 11 years. A period of exceptionally many sunspots is a sunspot maximum. Conversely, the Sun is almost devoid of sunspots at a sunspot minimum.
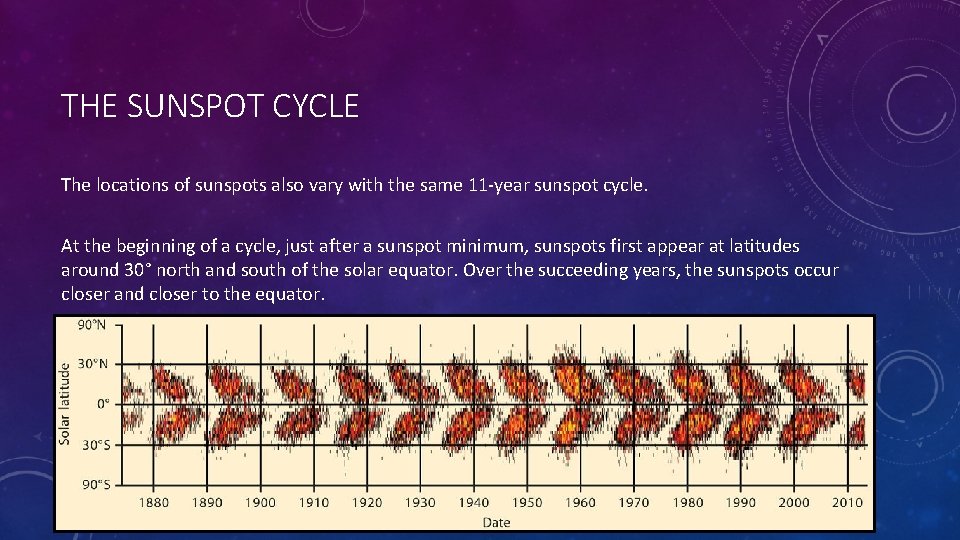
THE SUNSPOT CYCLE The locations of sunspots also vary with the same 11 -year sunspot cycle. At the beginning of a cycle, just after a sunspot minimum, sunspots first appear at latitudes around 30° north and south of the solar equator. Over the succeeding years, the sunspots occur closer and closer to the equator.
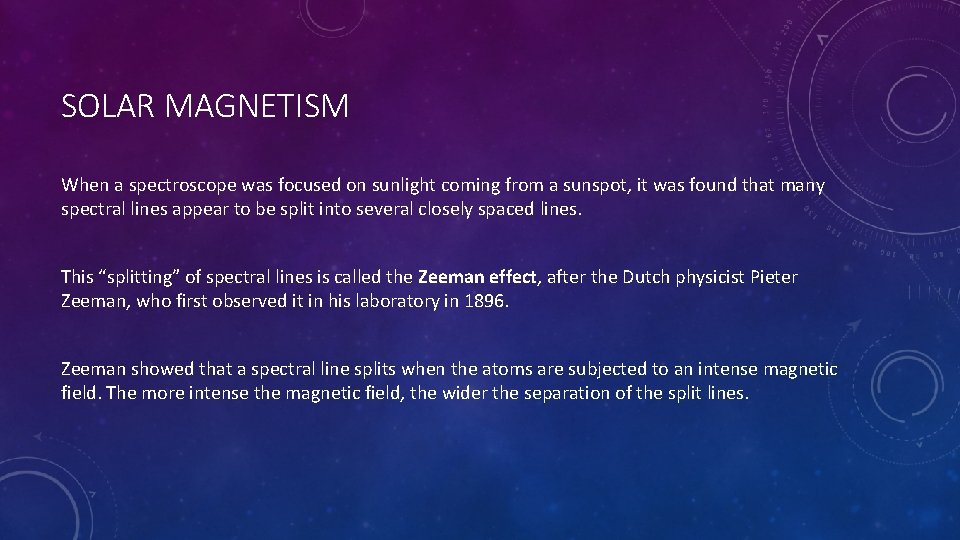
SOLAR MAGNETISM When a spectroscope was focused on sunlight coming from a sunspot, it was found that many spectral lines appear to be split into several closely spaced lines. This “splitting” of spectral lines is called the Zeeman effect, after the Dutch physicist Pieter Zeeman, who first observed it in his laboratory in 1896. Zeeman showed that a spectral line splits when the atoms are subjected to an intense magnetic field. The more intense the magnetic field, the wider the separation of the split lines.
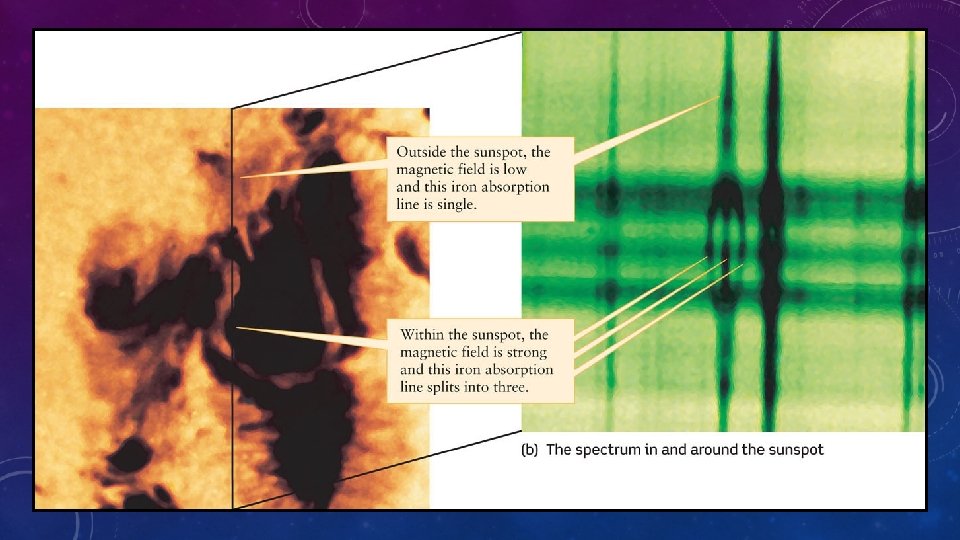
SOLAR MAGNETISM
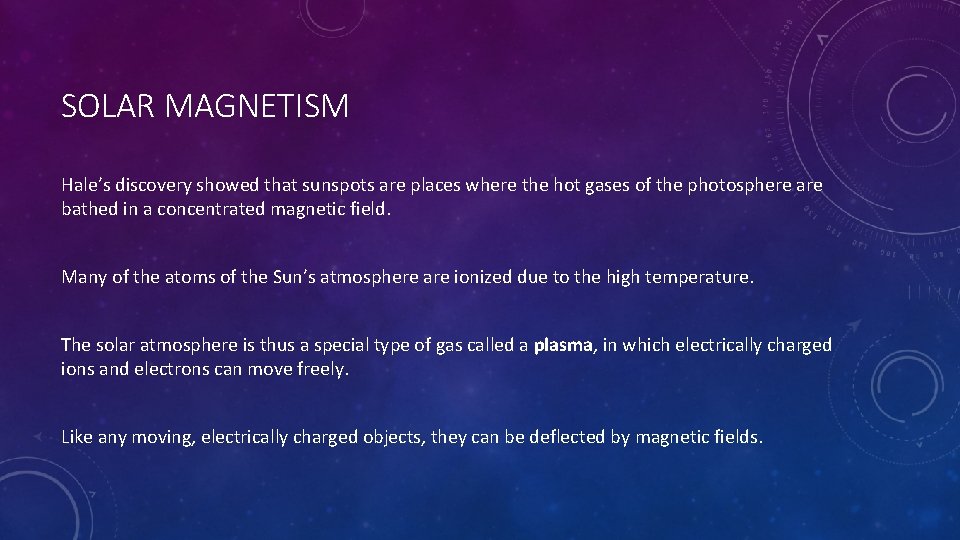
SOLAR MAGNETISM Hale’s discovery showed that sunspots are places where the hot gases of the photosphere are bathed in a concentrated magnetic field. Many of the atoms of the Sun’s atmosphere are ionized due to the high temperature. The solar atmosphere is thus a special type of gas called a plasma, in which electrically charged ions and electrons can move freely. Like any moving, electrically charged objects, they can be deflected by magnetic fields.
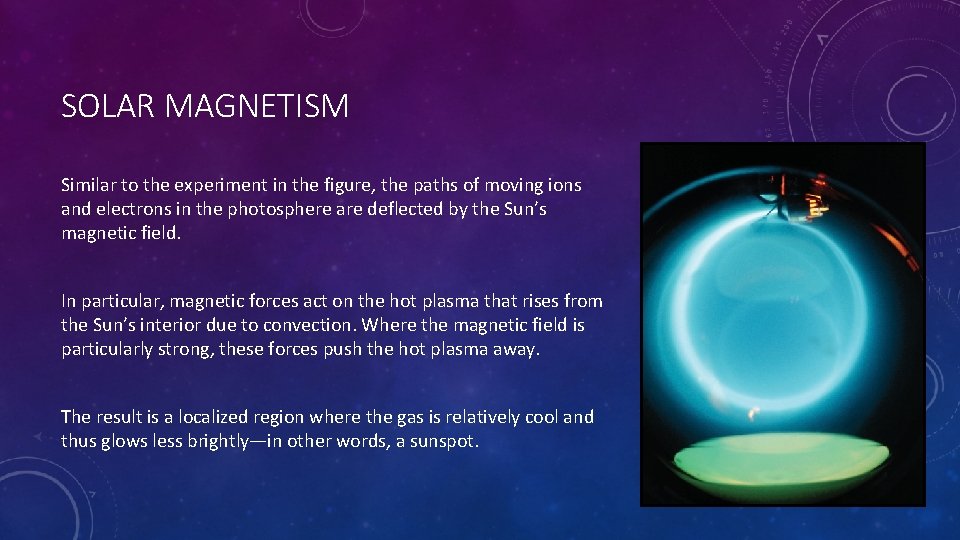
SOLAR MAGNETISM Similar to the experiment in the figure, the paths of moving ions and electrons in the photosphere are deflected by the Sun’s magnetic field. In particular, magnetic forces act on the hot plasma that rises from the Sun’s interior due to convection. Where the magnetic field is particularly strong, these forces push the hot plasma away. The result is a localized region where the gas is relatively cool and thus glows less brightly—in other words, a sunspot.
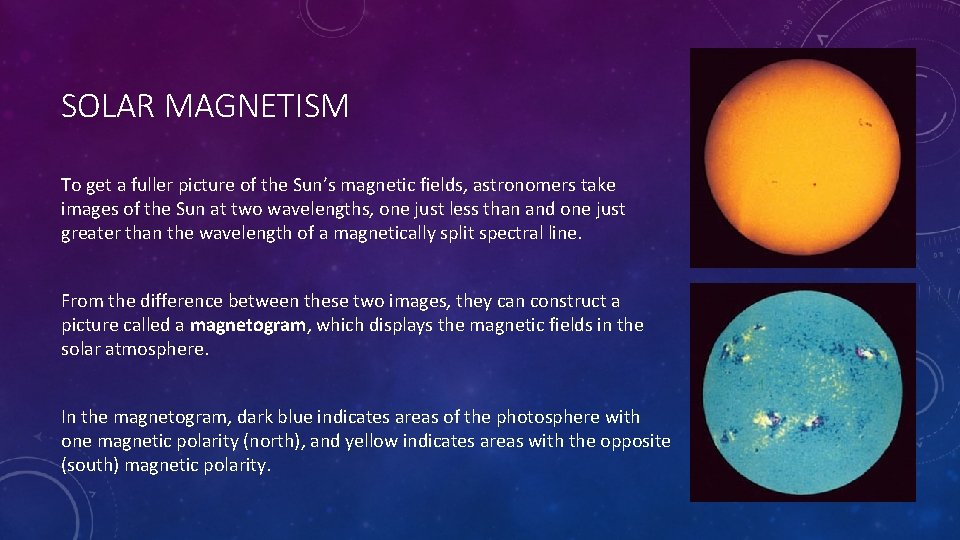
SOLAR MAGNETISM To get a fuller picture of the Sun’s magnetic fields, astronomers take images of the Sun at two wavelengths, one just less than and one just greater than the wavelength of a magnetically split spectral line. From the difference between these two images, they can construct a picture called a magnetogram, which displays the magnetic fields in the solar atmosphere. In the magnetogram, dark blue indicates areas of the photosphere with one magnetic polarity (north), and yellow indicates areas with the opposite (south) magnetic polarity.
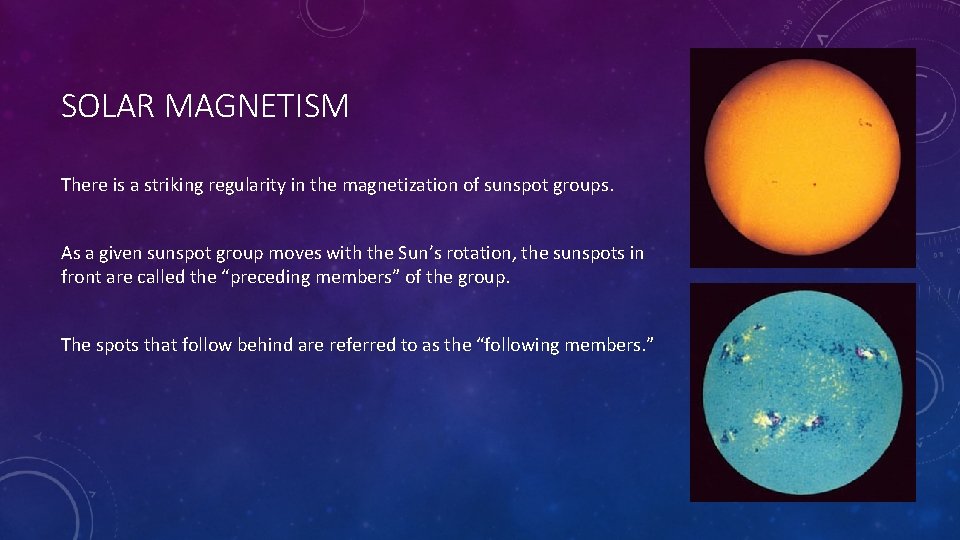
SOLAR MAGNETISM There is a striking regularity in the magnetization of sunspot groups. As a given sunspot group moves with the Sun’s rotation, the sunspots in front are called the “preceding members” of the group. The spots that follow behind are referred to as the “following members. ”
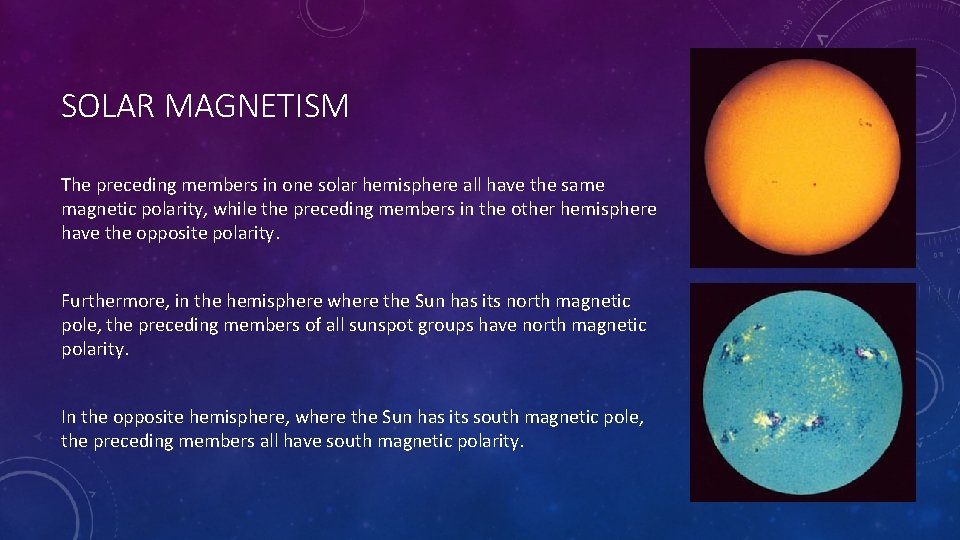
SOLAR MAGNETISM The preceding members in one solar hemisphere all have the same magnetic polarity, while the preceding members in the other hemisphere have the opposite polarity. Furthermore, in the hemisphere where the Sun has its north magnetic pole, the preceding members of all sunspot groups have north magnetic polarity. In the opposite hemisphere, where the Sun has its south magnetic pole, the preceding members all have south magnetic polarity.
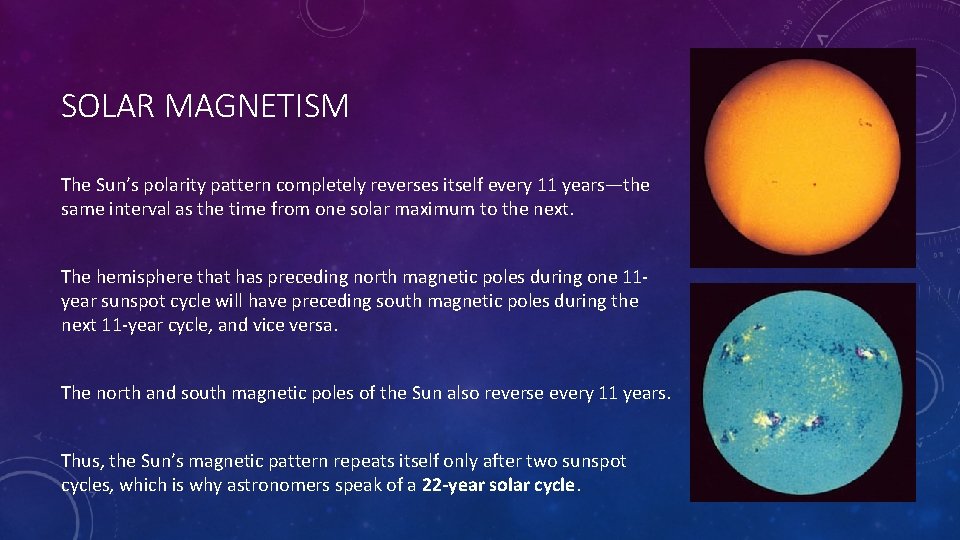
SOLAR MAGNETISM The Sun’s polarity pattern completely reverses itself every 11 years—the same interval as the time from one solar maximum to the next. The hemisphere that has preceding north magnetic poles during one 11 year sunspot cycle will have preceding south magnetic poles during the next 11 -year cycle, and vice versa. The north and south magnetic poles of the Sun also reverse every 11 years. Thus, the Sun’s magnetic pattern repeats itself only after two sunspot cycles, which is why astronomers speak of a 22 -year solar cycle.
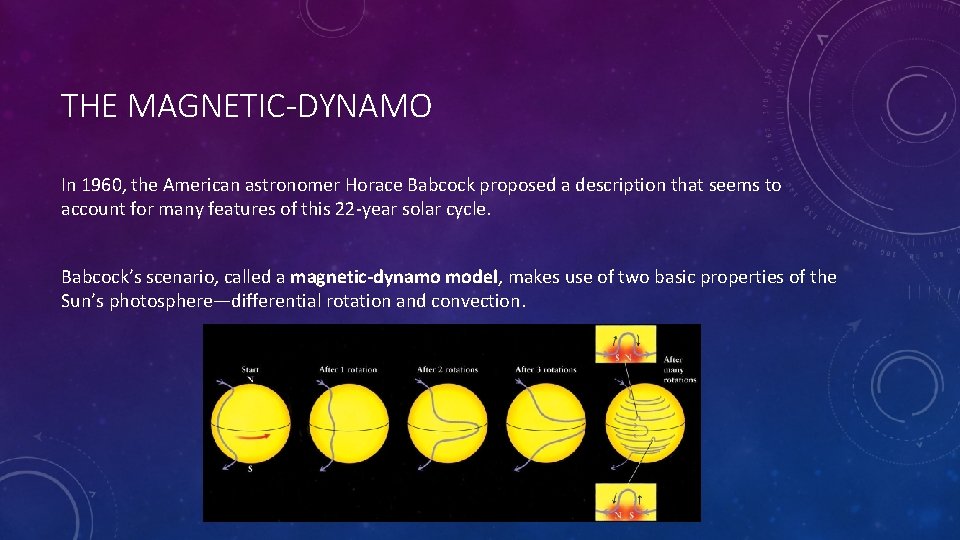
THE MAGNETIC-DYNAMO In 1960, the American astronomer Horace Babcock proposed a description that seems to account for many features of this 22 -year solar cycle. Babcock’s scenario, called a magnetic-dynamo model, makes use of two basic properties of the Sun’s photosphere—differential rotation and convection.
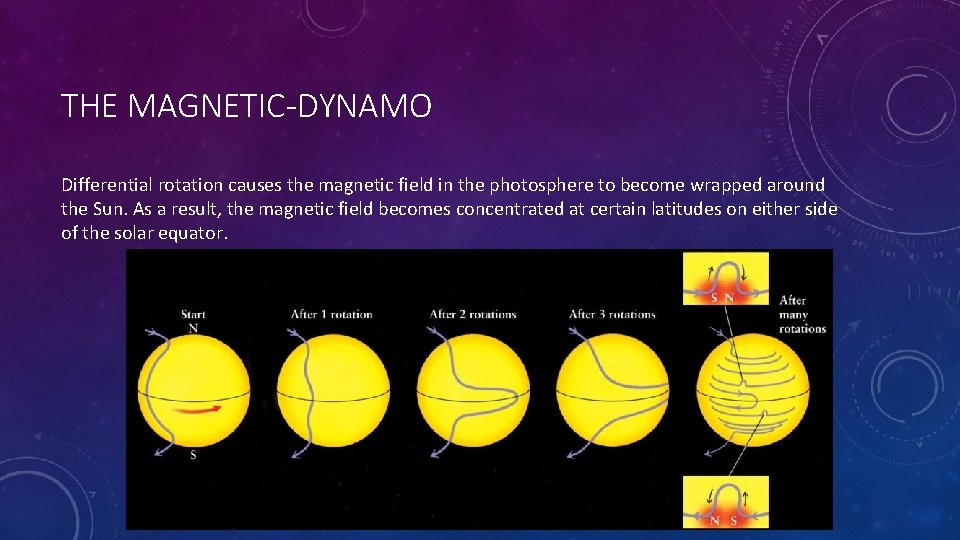
THE MAGNETIC-DYNAMO Differential rotation causes the magnetic field in the photosphere to become wrapped around the Sun. As a result, the magnetic field becomes concentrated at certain latitudes on either side of the solar equator.
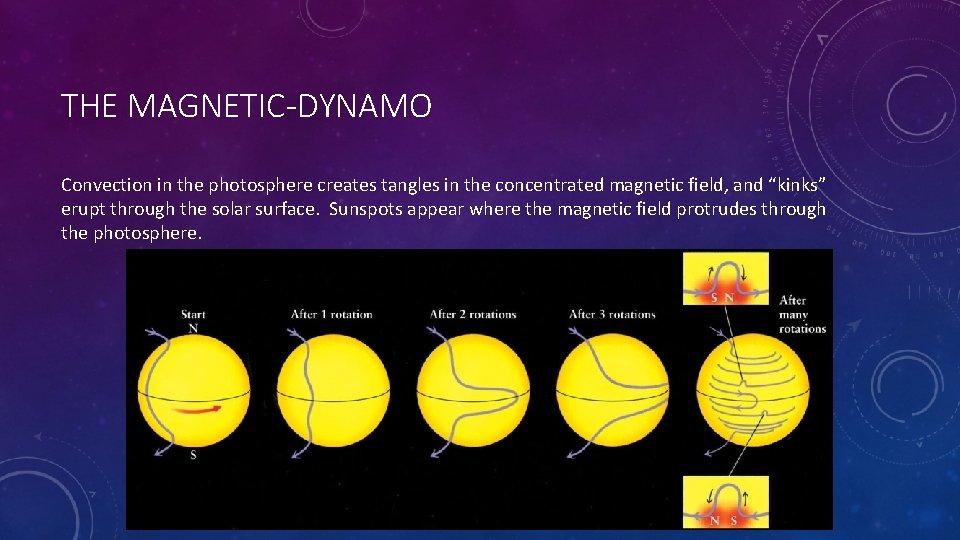
THE MAGNETIC-DYNAMO Convection in the photosphere creates tangles in the concentrated magnetic field, and “kinks” erupt through the solar surface. Sunspots appear where the magnetic field protrudes through the photosphere.
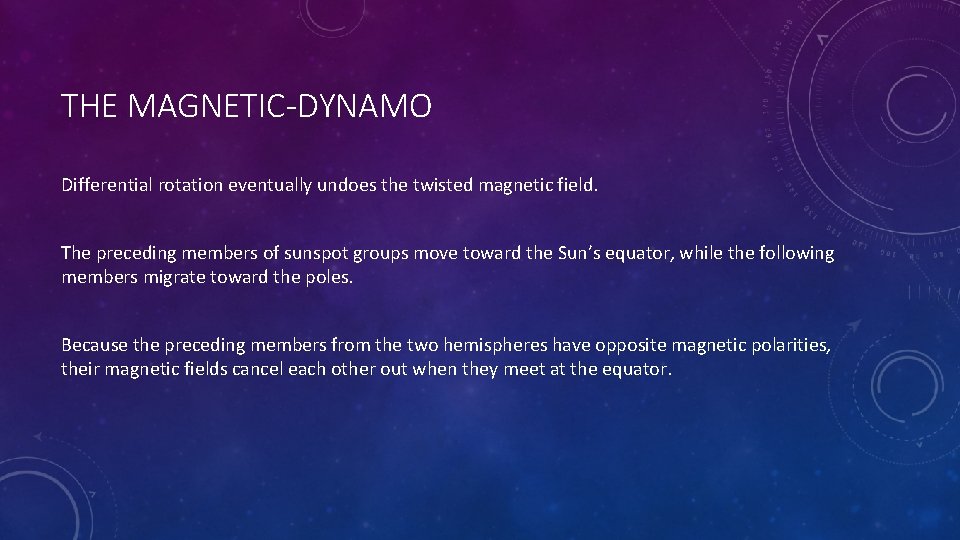
THE MAGNETIC-DYNAMO Differential rotation eventually undoes the twisted magnetic field. The preceding members of sunspot groups move toward the Sun’s equator, while the following members migrate toward the poles. Because the preceding members from the two hemispheres have opposite magnetic polarities, their magnetic fields cancel each other out when they meet at the equator.
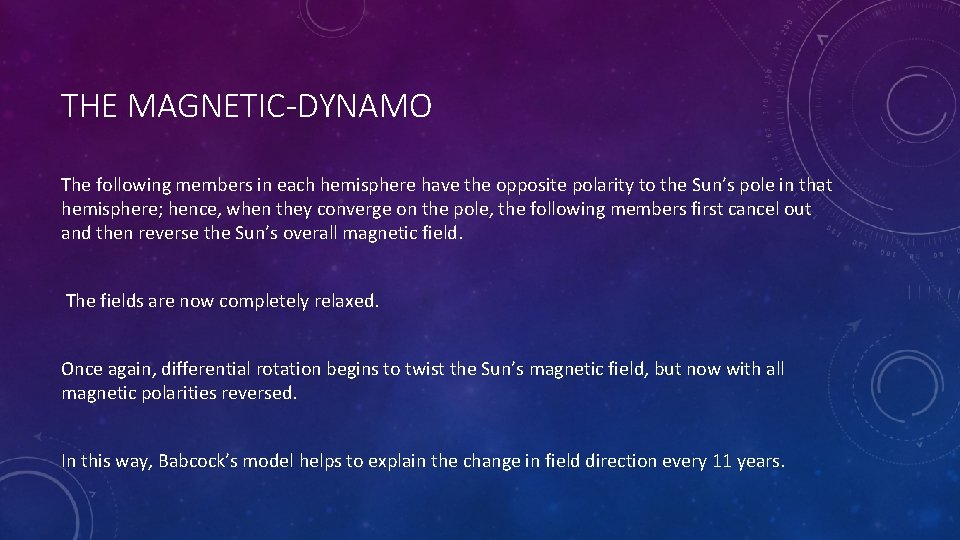
THE MAGNETIC-DYNAMO The following members in each hemisphere have the opposite polarity to the Sun’s pole in that hemisphere; hence, when they converge on the pole, the following members first cancel out and then reverse the Sun’s overall magnetic field. The fields are now completely relaxed. Once again, differential rotation begins to twist the Sun’s magnetic field, but now with all magnetic polarities reversed. In this way, Babcock’s model helps to explain the change in field direction every 11 years.
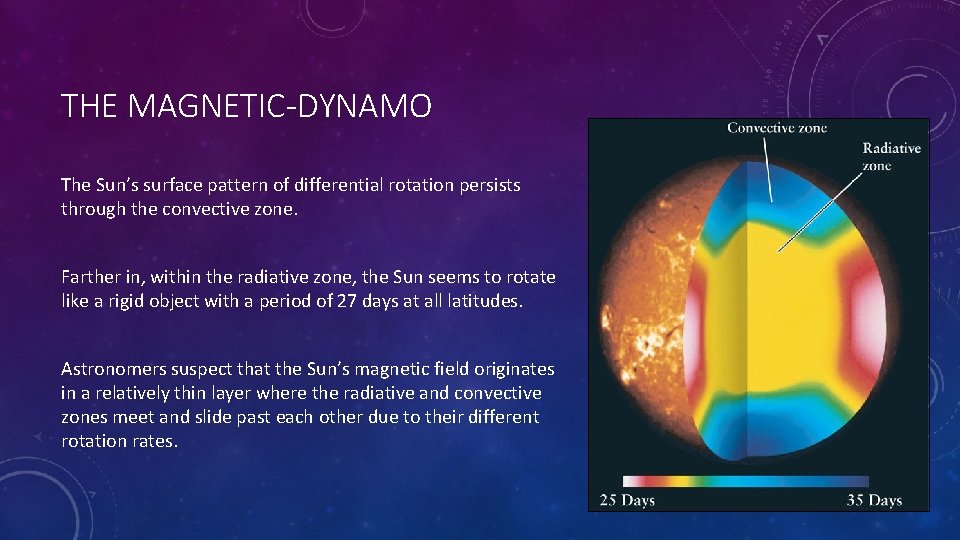
THE MAGNETIC-DYNAMO The Sun’s surface pattern of differential rotation persists through the convective zone. Farther in, within the radiative zone, the Sun seems to rotate like a rigid object with a period of 27 days at all latitudes. Astronomers suspect that the Sun’s magnetic field originates in a relatively thin layer where the radiative and convective zones meet and slide past each other due to their different rotation rates.
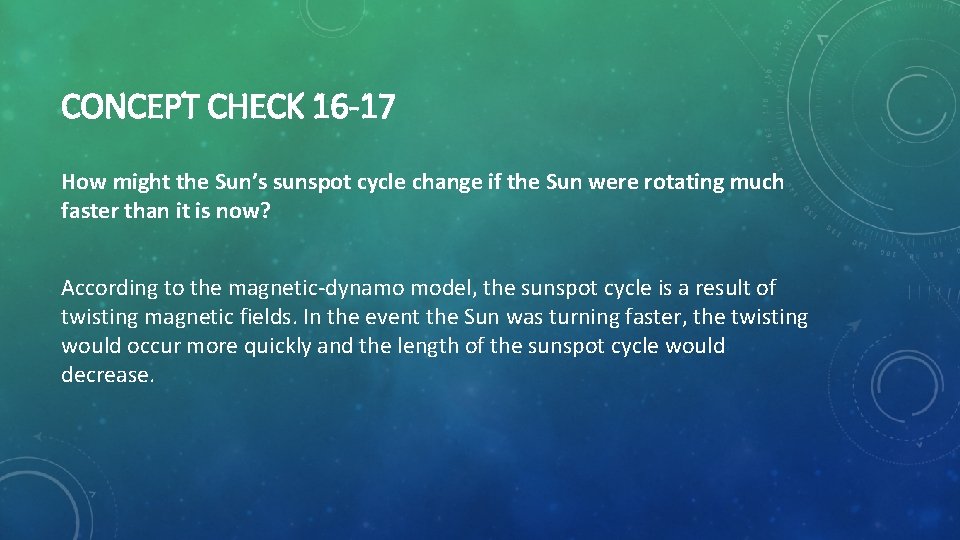
CONCEPT CHECK 16 -17 How might the Sun’s sunspot cycle change if the Sun were rotating much faster than it is now? According to the magnetic-dynamo model, the sunspot cycle is a result of twisting magnetic fields. In the event the Sun was turning faster, the twisting would occur more quickly and the length of the sunspot cycle would decrease.
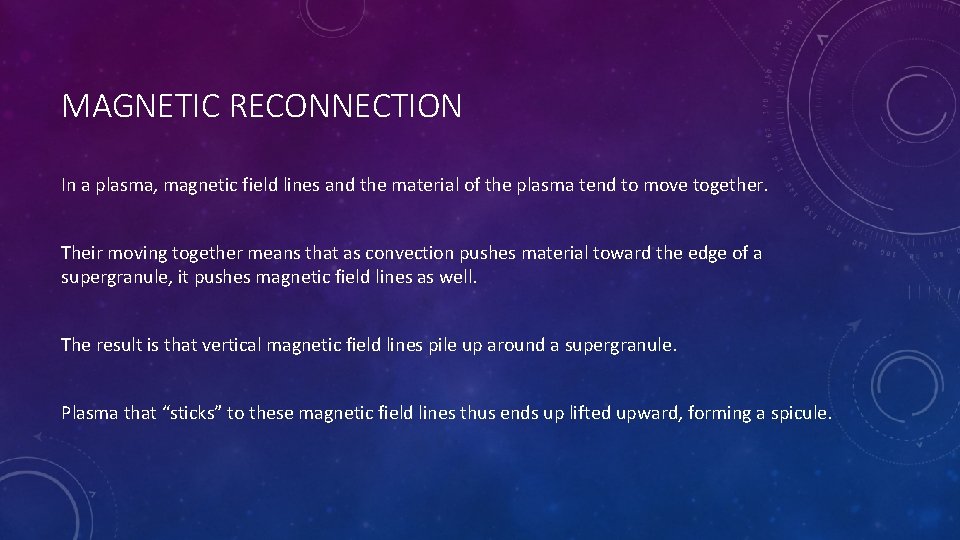
MAGNETIC RECONNECTION In a plasma, magnetic field lines and the material of the plasma tend to move together. Their moving together means that as convection pushes material toward the edge of a supergranule, it pushes magnetic field lines as well. The result is that vertical magnetic field lines pile up around a supergranule. Plasma that “sticks” to these magnetic field lines thus ends up lifted upward, forming a spicule.
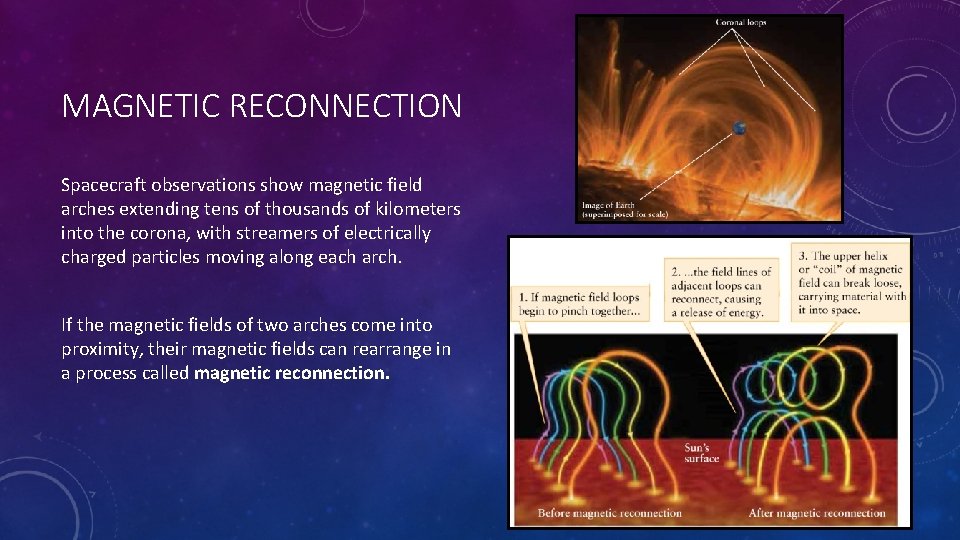
MAGNETIC RECONNECTION Spacecraft observations show magnetic field arches extending tens of thousands of kilometers into the corona, with streamers of electrically charged particles moving along each arch. If the magnetic fields of two arches come into proximity, their magnetic fields can rearrange in a process called magnetic reconnection.
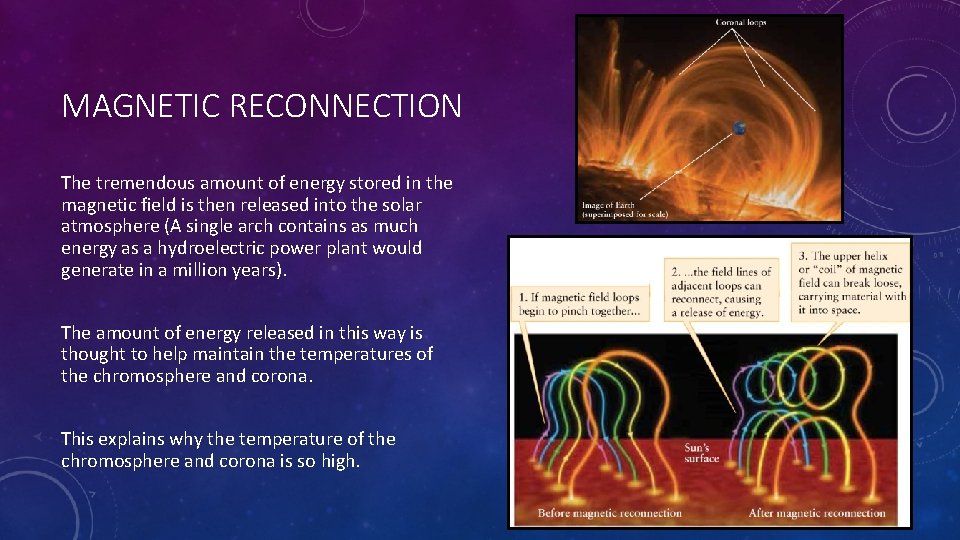
MAGNETIC RECONNECTION The tremendous amount of energy stored in the magnetic field is then released into the solar atmosphere (A single arch contains as much energy as a hydroelectric power plant would generate in a million years). The amount of energy released in this way is thought to help maintain the temperatures of the chromosphere and corona. This explains why the temperature of the chromosphere and corona is so high.
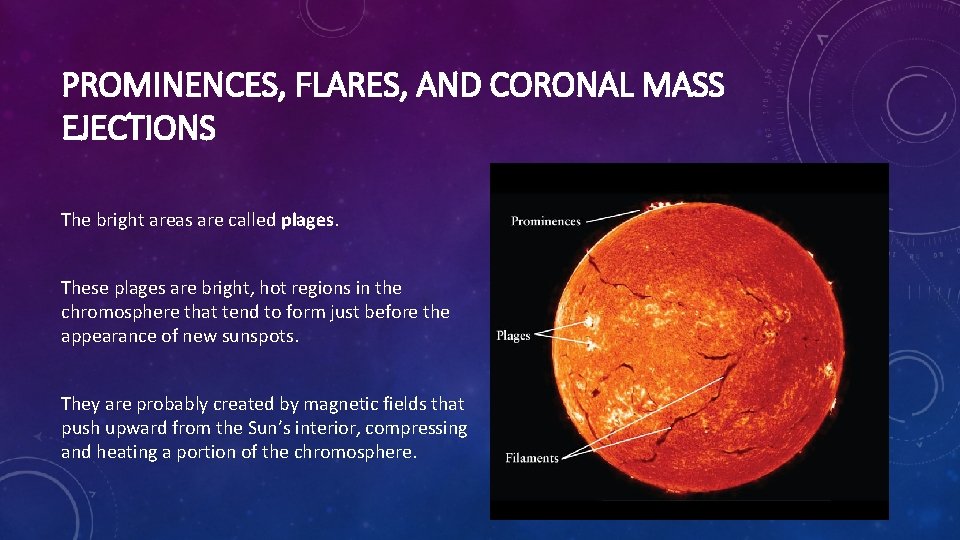
PROMINENCES, FLARES, AND CORONAL MASS EJECTIONS The bright areas are called plages. These plages are bright, hot regions in the chromosphere that tend to form just before the appearance of new sunspots. They are probably created by magnetic fields that push upward from the Sun’s interior, compressing and heating a portion of the chromosphere.
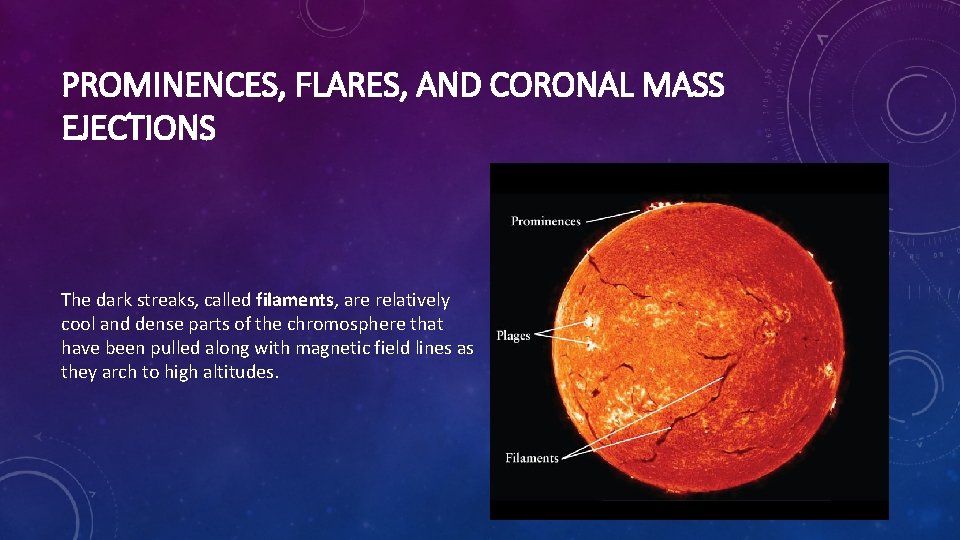
PROMINENCES, FLARES, AND CORONAL MASS EJECTIONS The dark streaks, called filaments, are relatively cool and dense parts of the chromosphere that have been pulled along with magnetic field lines as they arch to high altitudes.
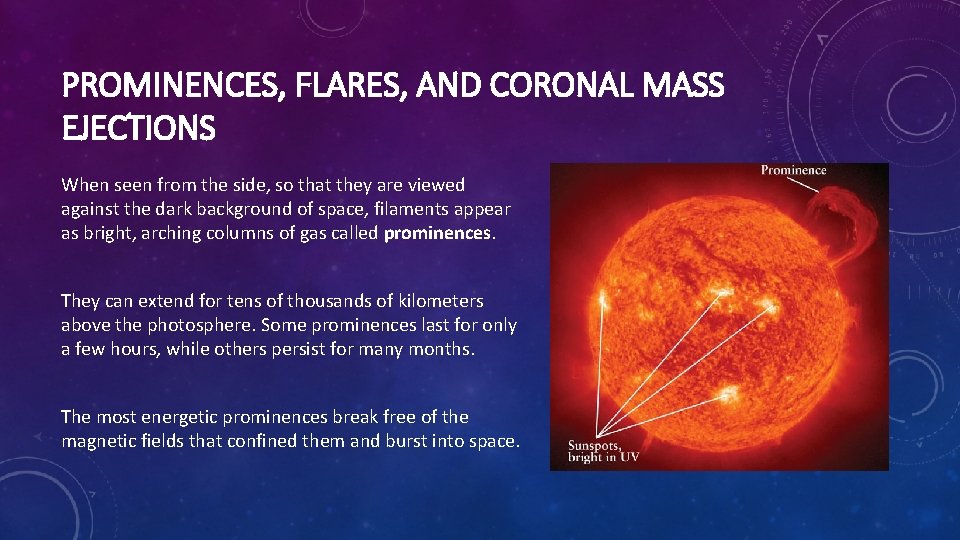
PROMINENCES, FLARES, AND CORONAL MASS EJECTIONS When seen from the side, so that they are viewed against the dark background of space, filaments appear as bright, arching columns of gas called prominences. They can extend for tens of thousands of kilometers above the photosphere. Some prominences last for only a few hours, while others persist for many months. The most energetic prominences break free of the magnetic fields that confined them and burst into space.
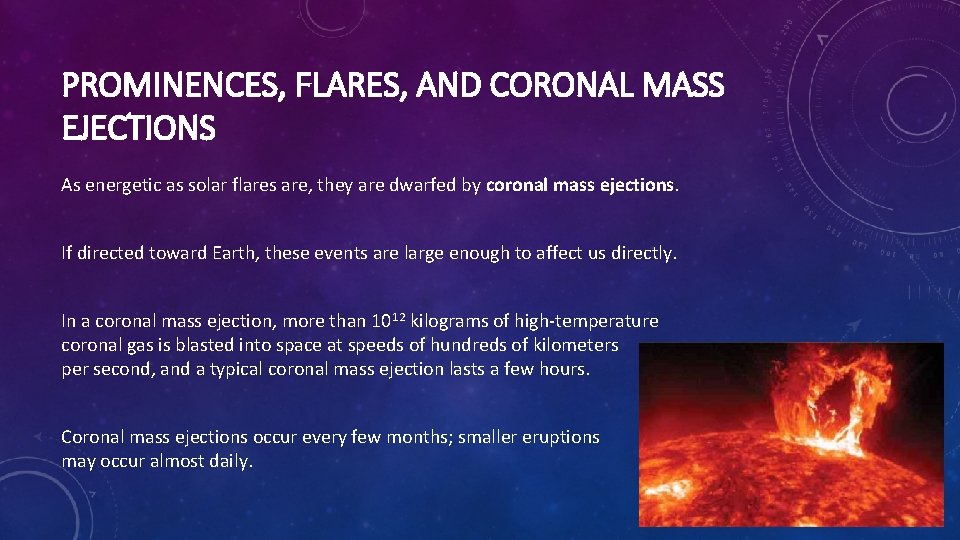
PROMINENCES, FLARES, AND CORONAL MASS EJECTIONS As energetic as solar flares are, they are dwarfed by coronal mass ejections. If directed toward Earth, these events are large enough to affect us directly. In a coronal mass ejection, more than 1012 kilograms of high-temperature coronal gas is blasted into space at speeds of hundreds of kilometers per second, and a typical coronal mass ejection lasts a few hours. Coronal mass ejections occur every few months; smaller eruptions may occur almost daily.
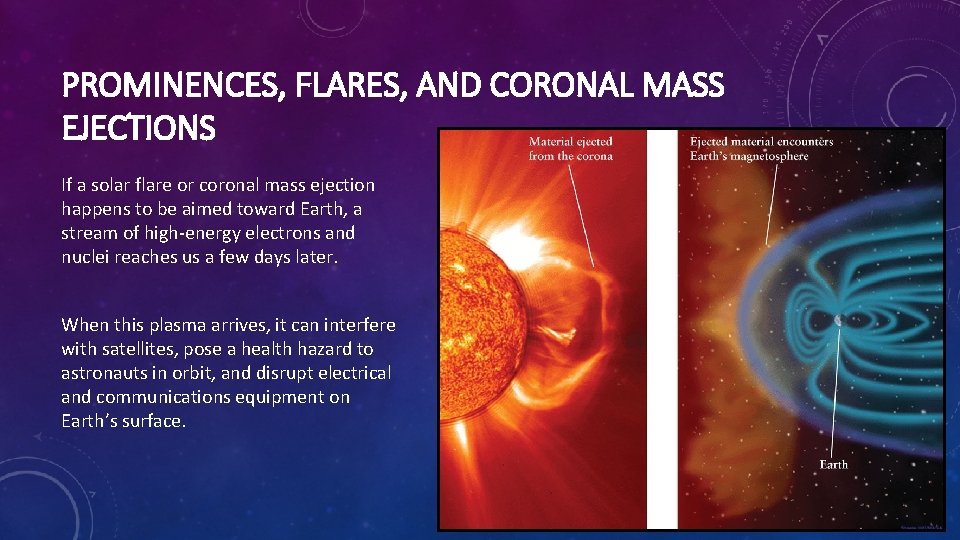
PROMINENCES, FLARES, AND CORONAL MASS EJECTIONS If a solar flare or coronal mass ejection happens to be aimed toward Earth, a stream of high-energy electrons and nuclei reaches us a few days later. When this plasma arrives, it can interfere with satellites, pose a health hazard to astronauts in orbit, and disrupt electrical and communications equipment on Earth’s surface.
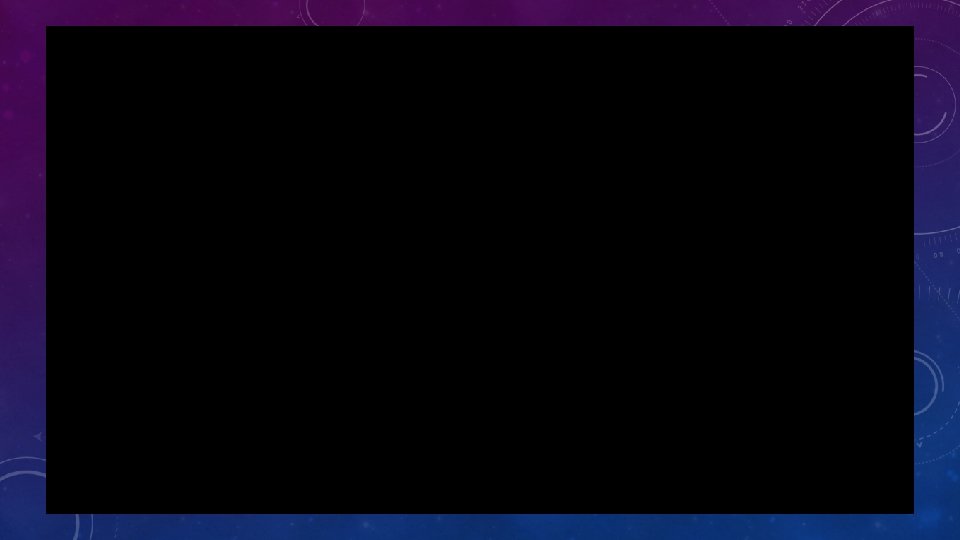
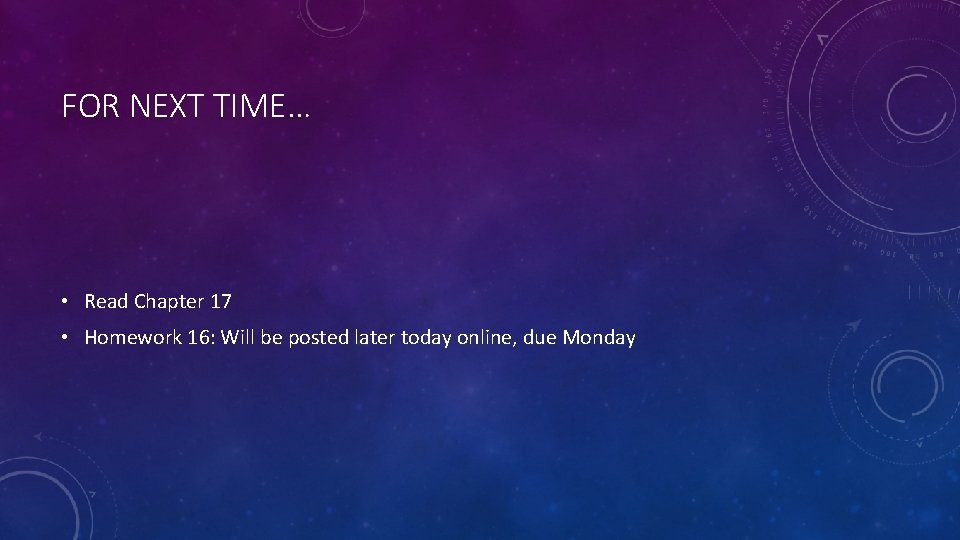
FOR NEXT TIME… • Read Chapter 17 • Homework 16: Will be posted later today online, due Monday
Jeremias 1414 falsos profetas
Learning astronomy by doing astronomy activity 1 answers
Learning astronomy by doing astronomy activity 7 answers
Learning astronomy by doing astronomy
Psci audit
Pharmaceutical supply chain initiative psci
Pharmaceutical supply chain initiative
The visible light we see from our sun comes from which part
Hát kết hợp bộ gõ cơ thể
Bổ thể
Tỉ lệ cơ thể trẻ em
Chó sói
Chụp phim tư thế worms-breton
Bài hát chúa yêu trần thế alleluia
Kể tên các môn thể thao
Thế nào là hệ số cao nhất
Các châu lục và đại dương trên thế giới
Công thức tính thế năng
Trời xanh đây là của chúng ta thể thơ
Mật thư anh em như thể tay chân
Làm thế nào để 102-1=99
độ dài liên kết
Các châu lục và đại dương trên thế giới
Thơ thất ngôn tứ tuyệt đường luật
Quá trình desamine hóa có thể tạo ra
Một số thể thơ truyền thống
Cái miệng nó xinh thế
Vẽ hình chiếu vuông góc của vật thể sau
Nguyên nhân của sự mỏi cơ sinh 8
đặc điểm cơ thể của người tối cổ
Thế nào là giọng cùng tên? *
Vẽ hình chiếu đứng bằng cạnh của vật thể
Fecboak
Thẻ vin
đại từ thay thế