Mechanical Design of Engine Parts P M V
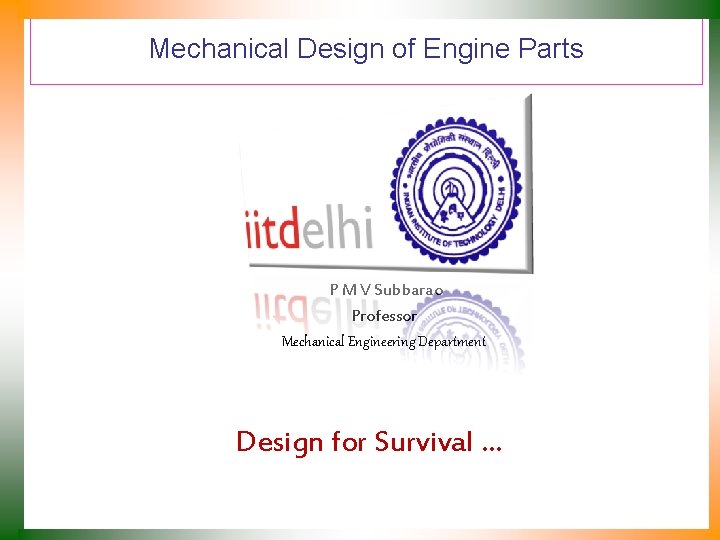
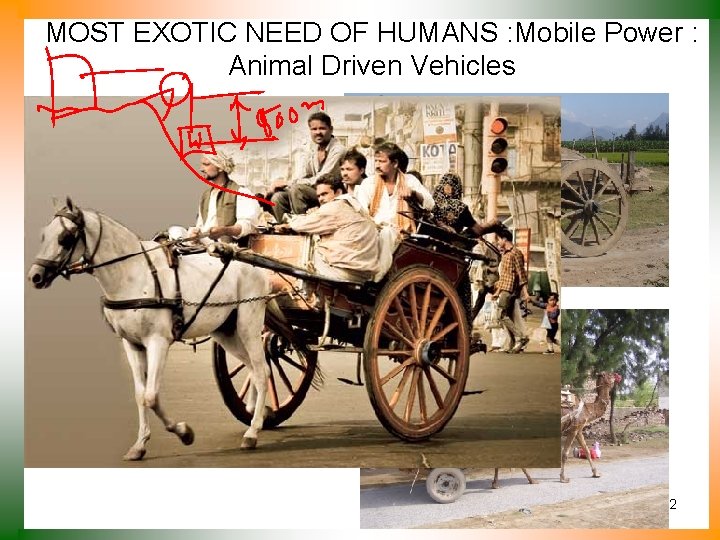
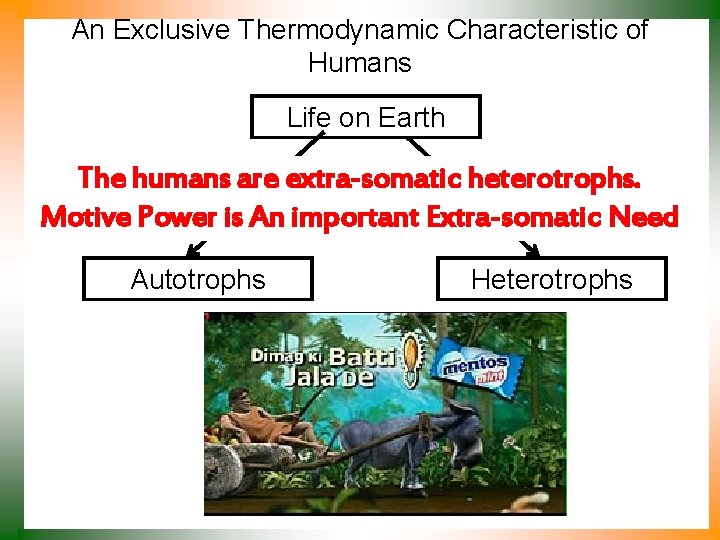
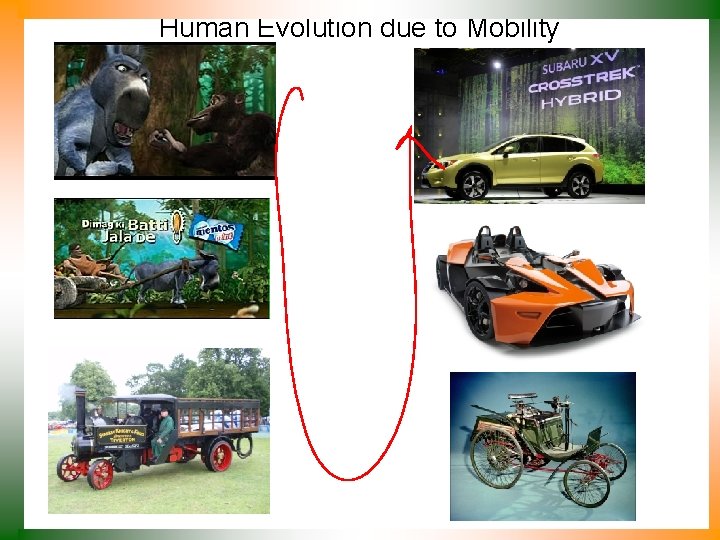
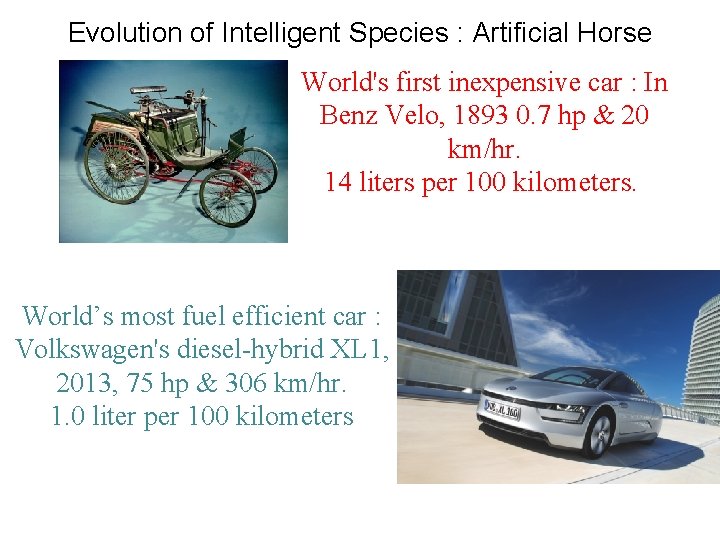
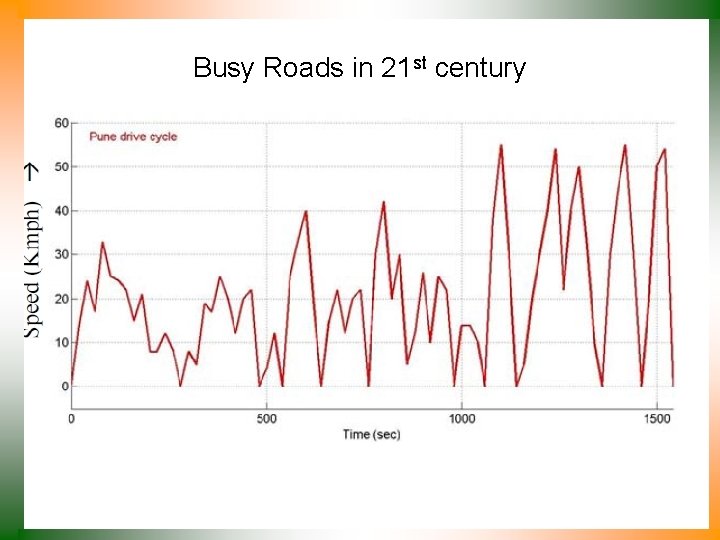
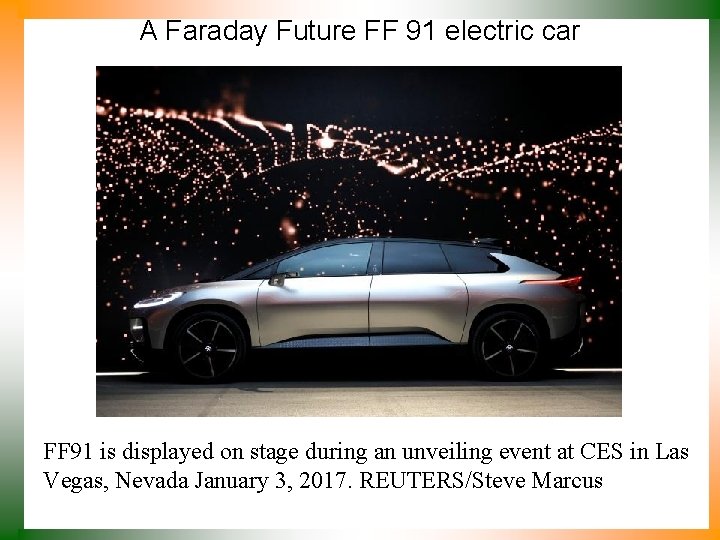
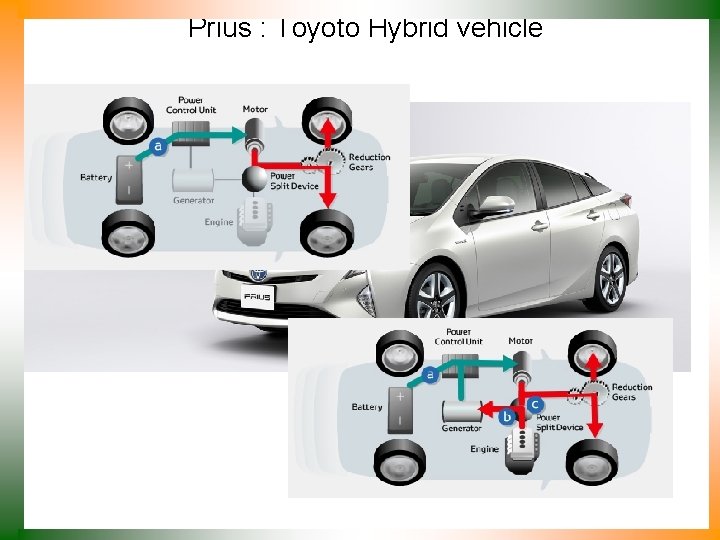
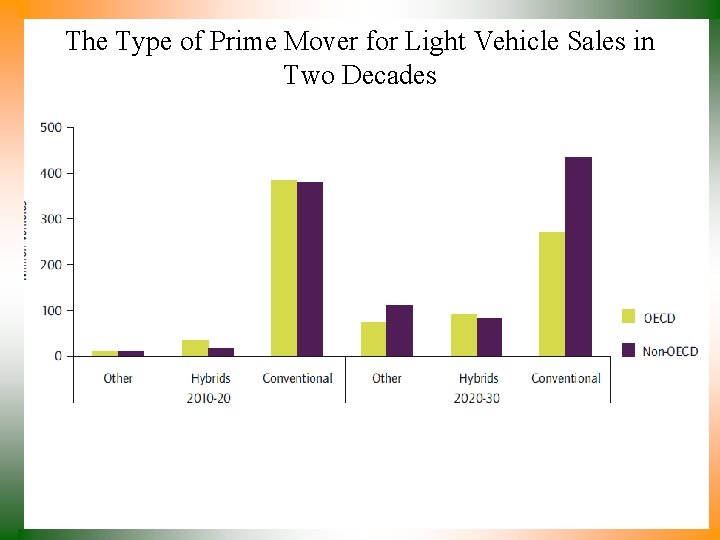
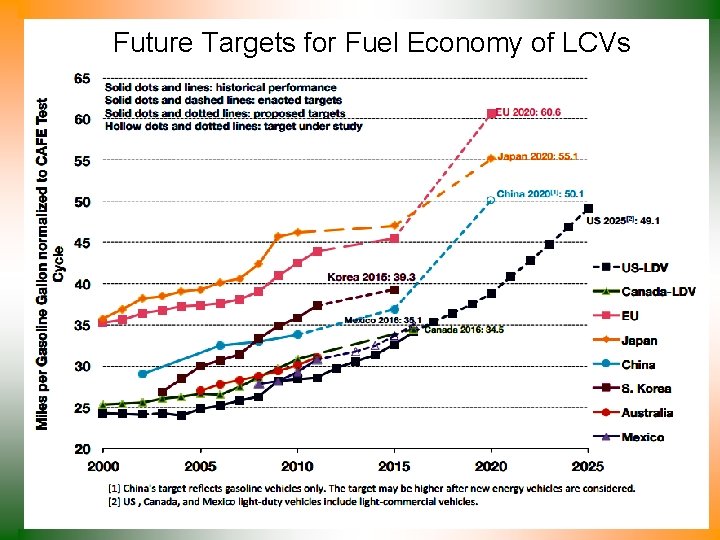
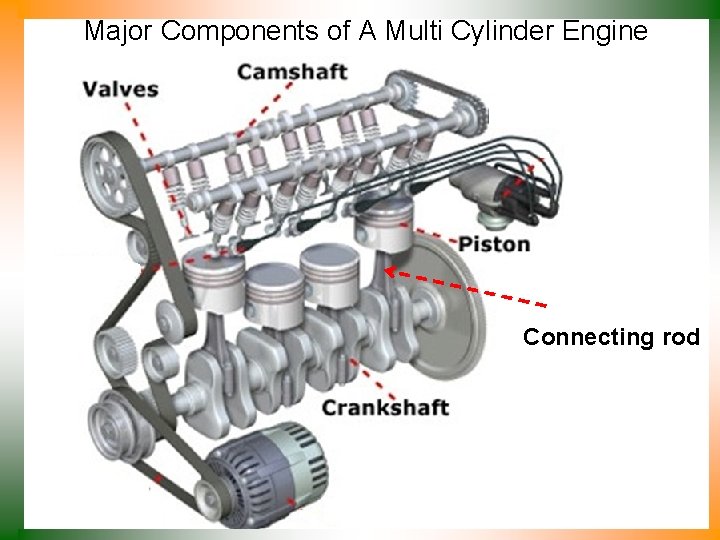
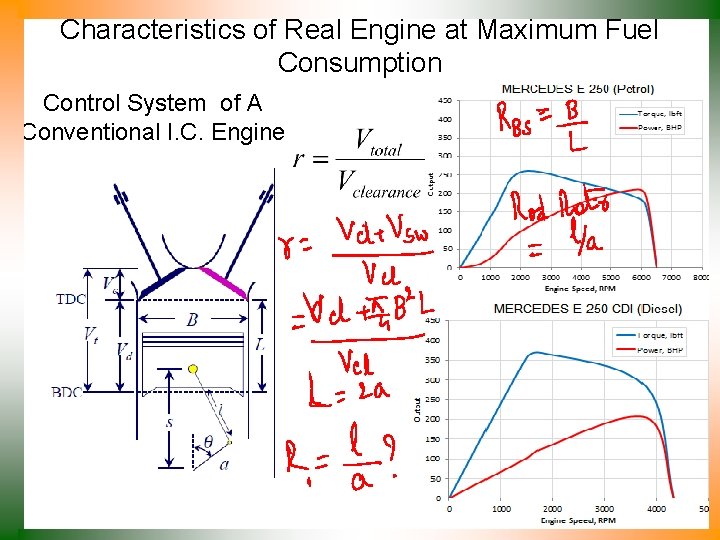
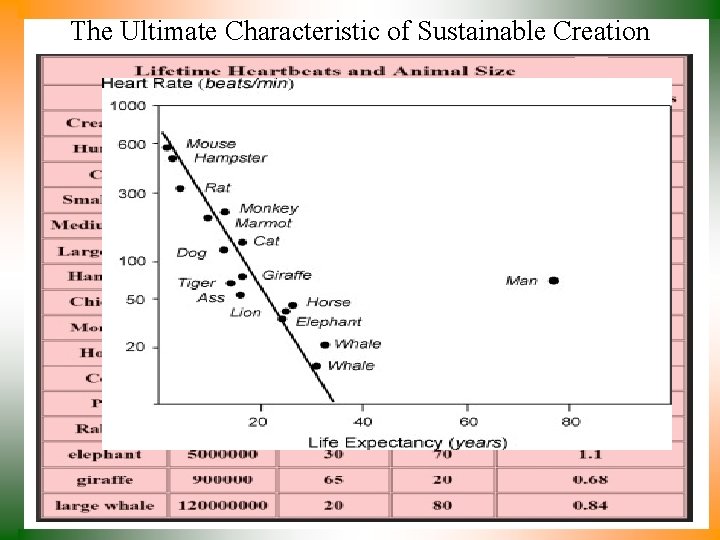
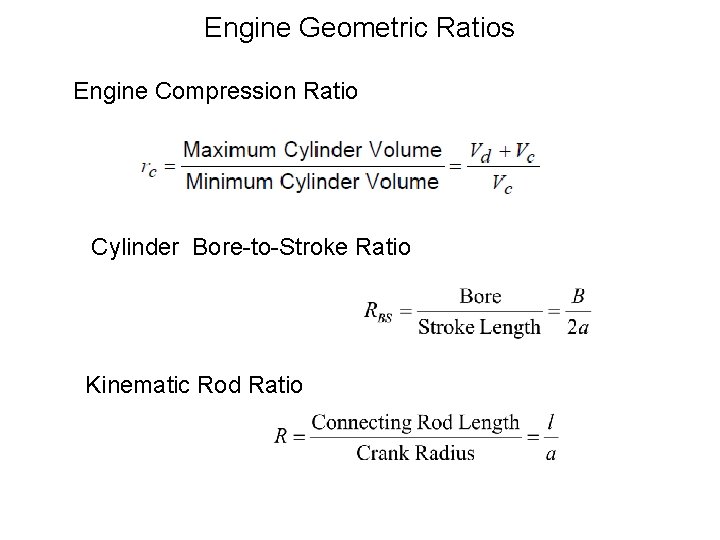
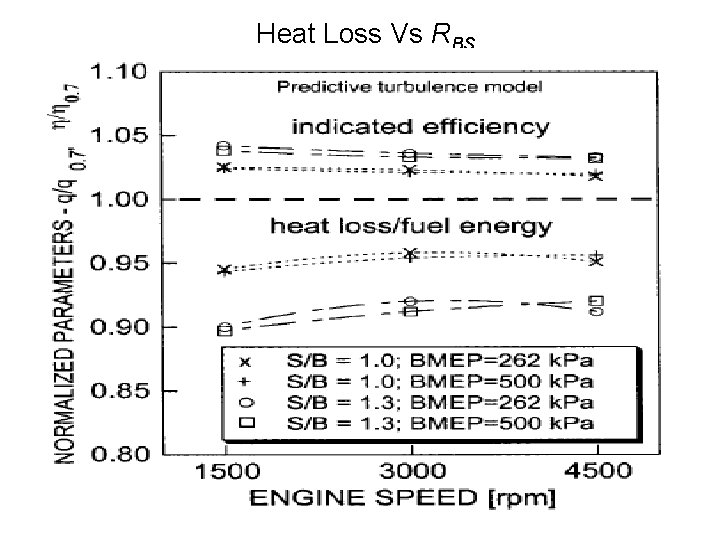
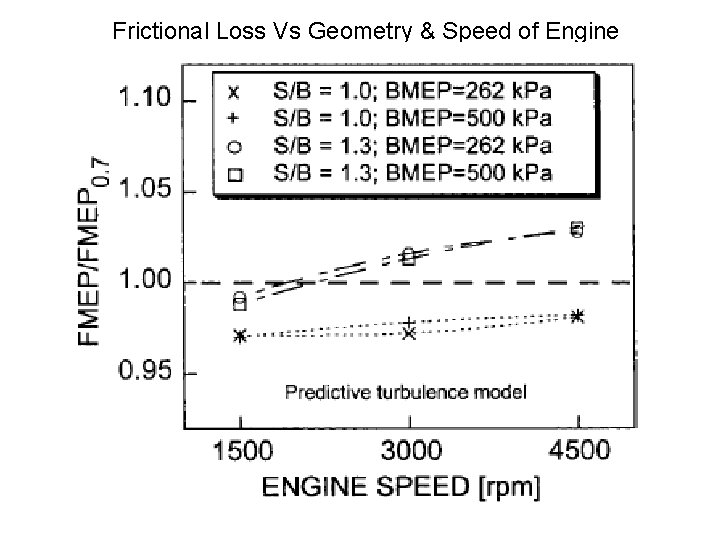
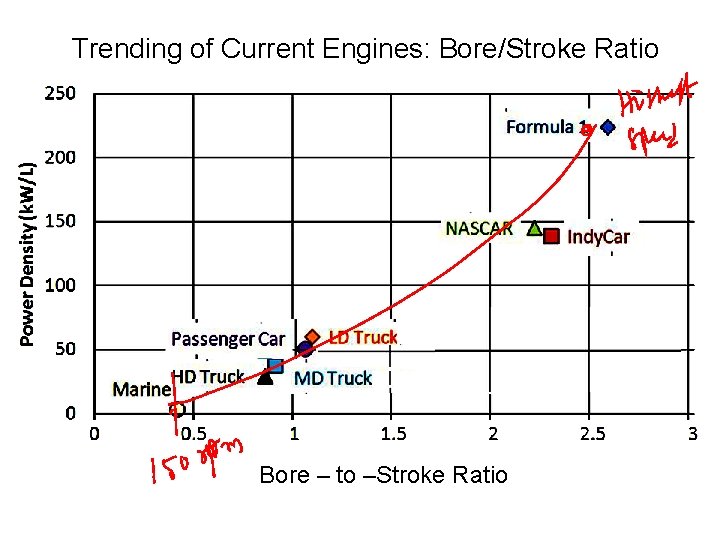
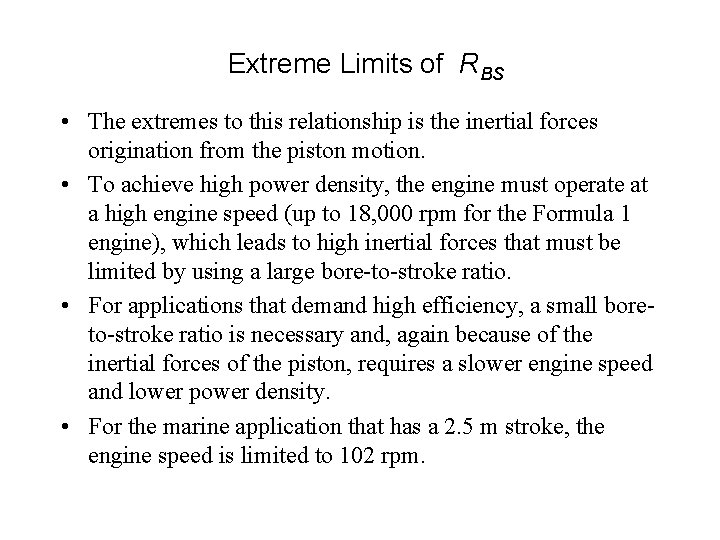
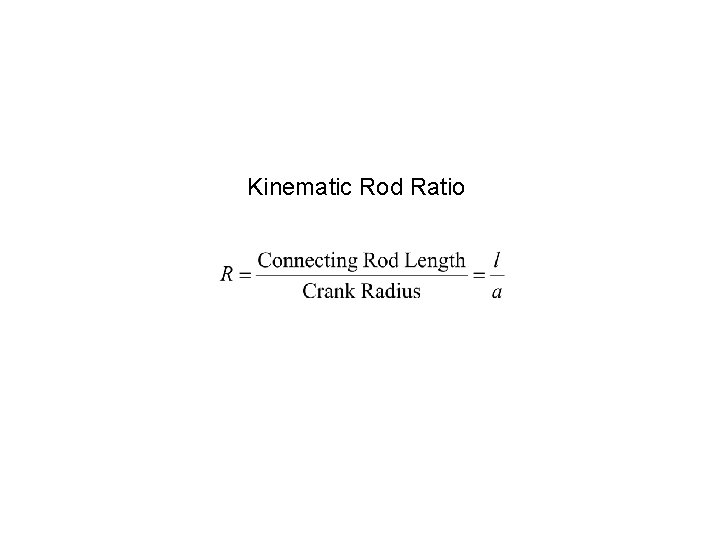
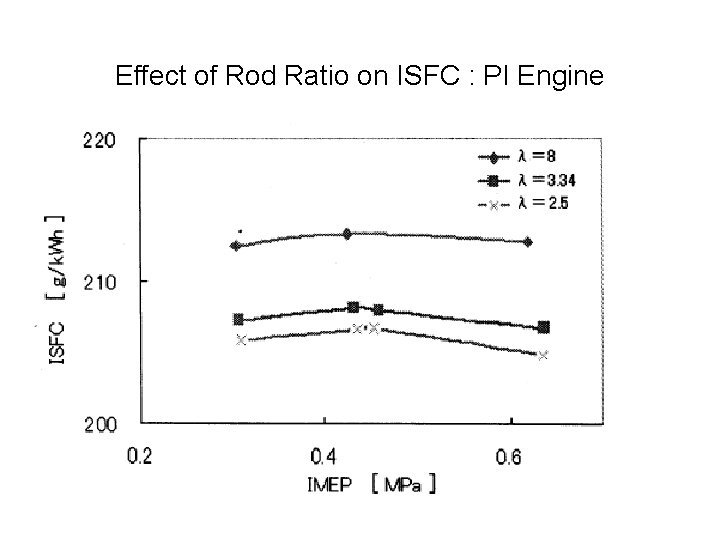
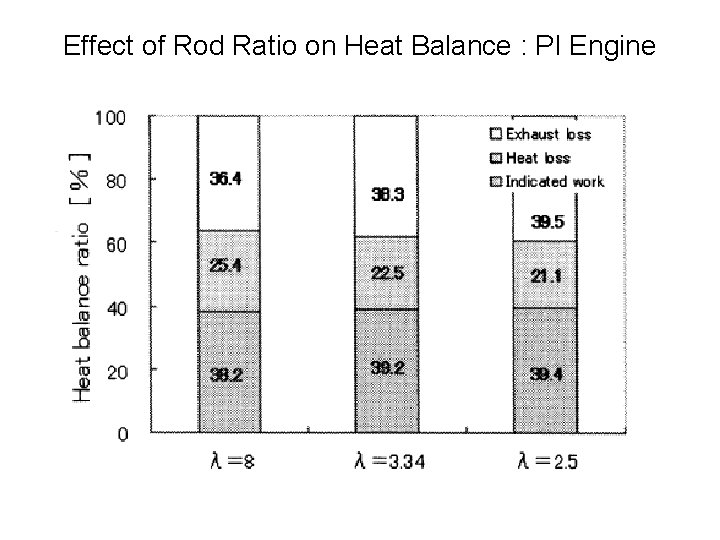
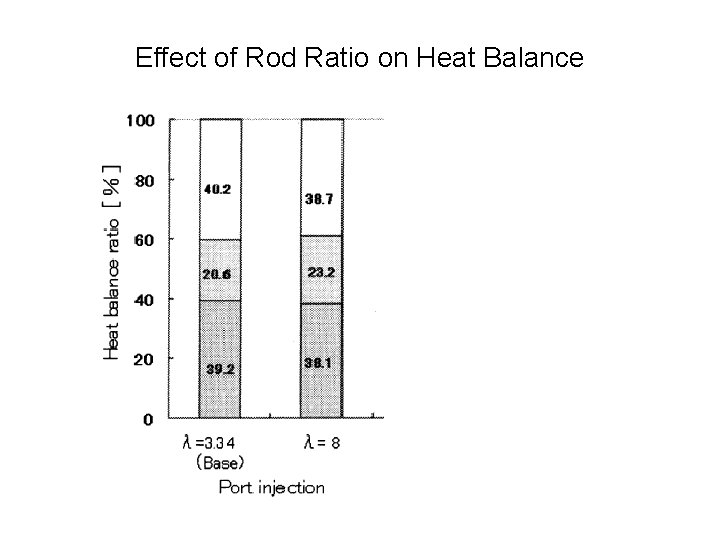
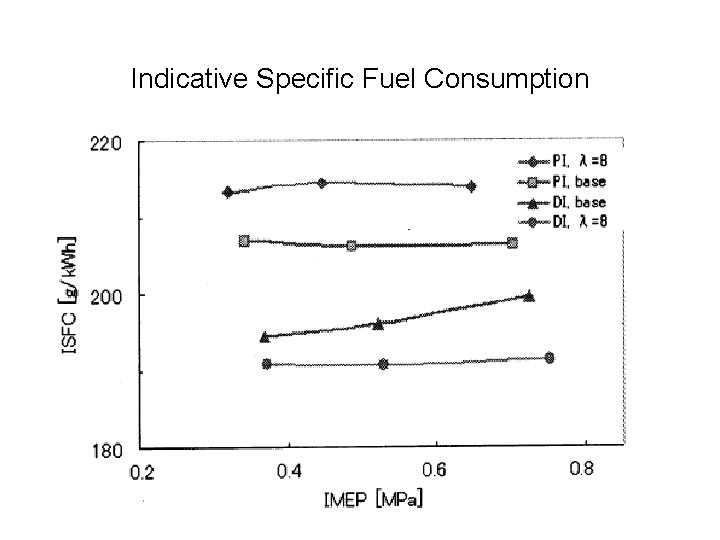
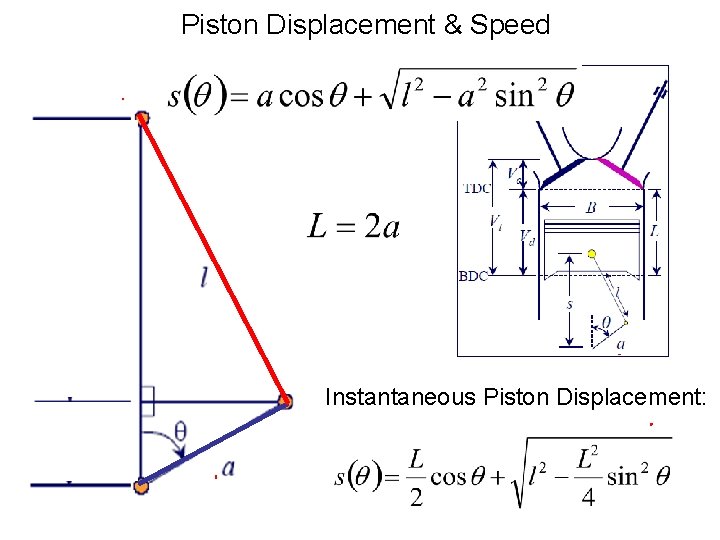
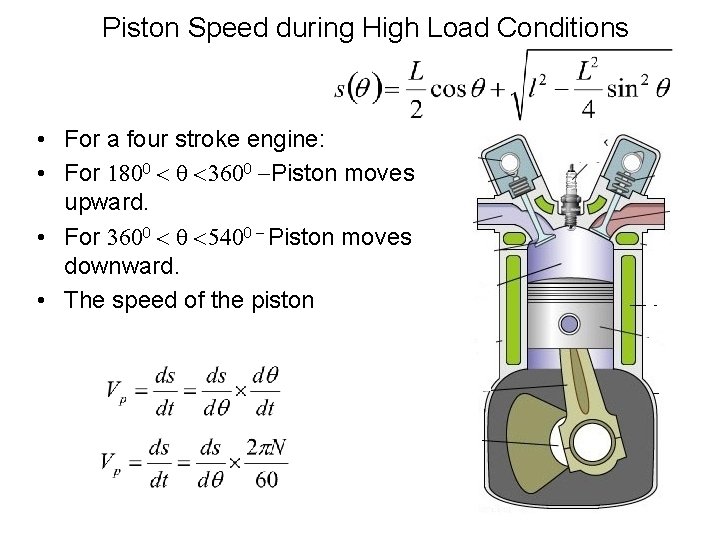
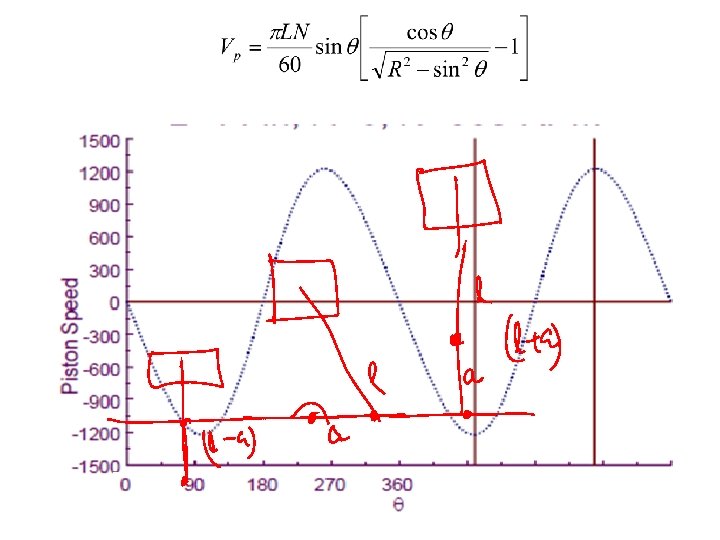
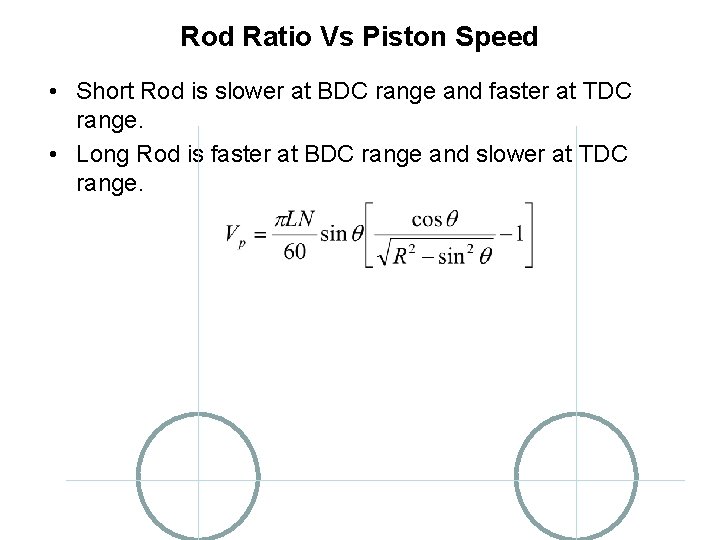
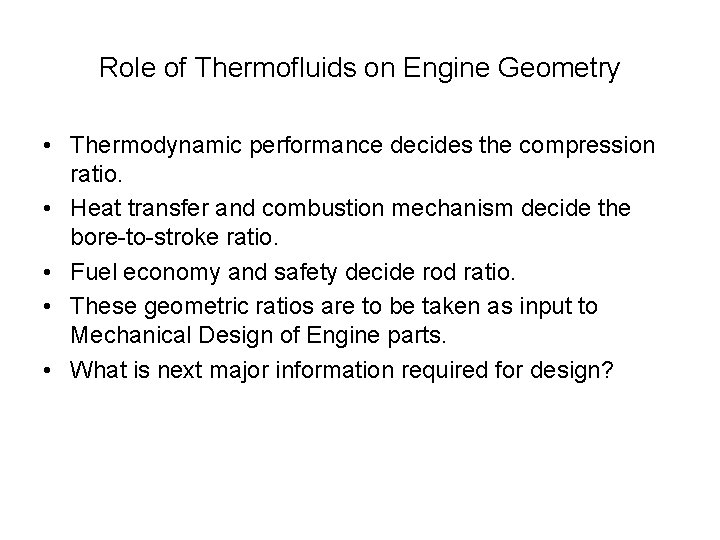
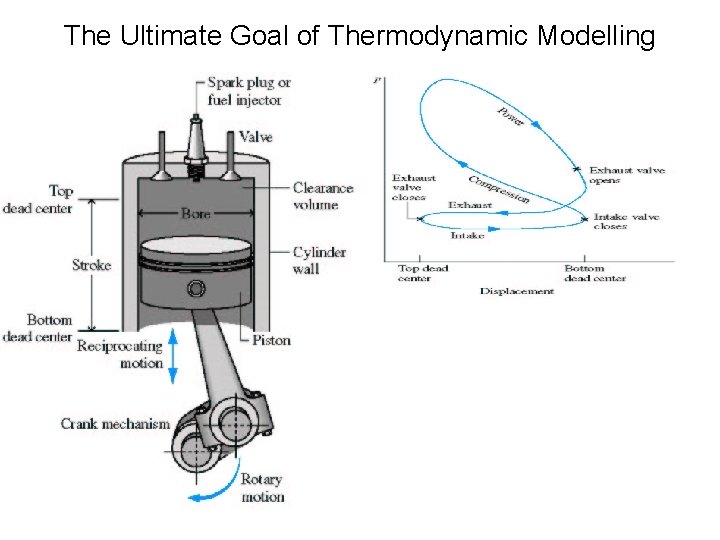
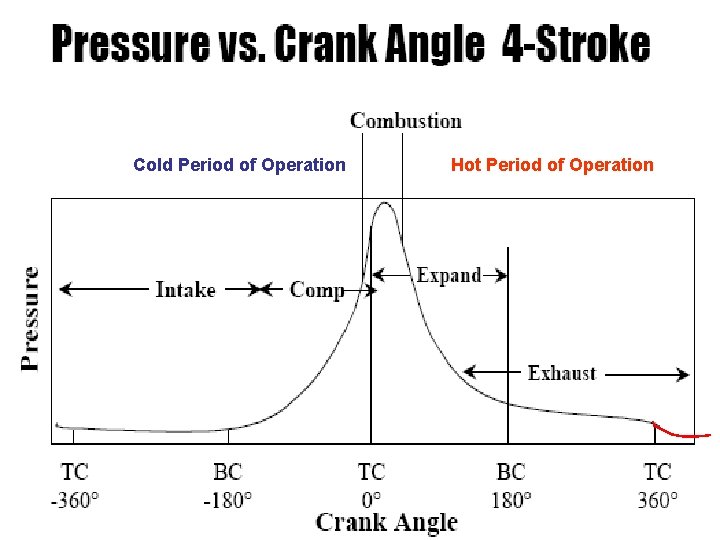
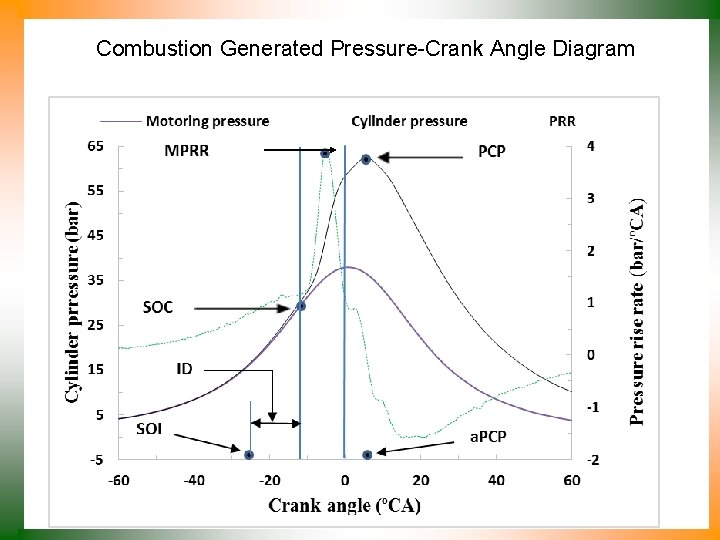
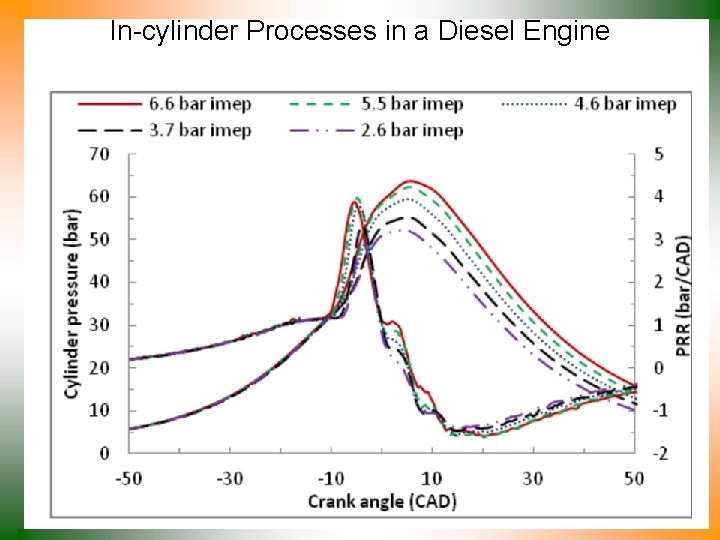
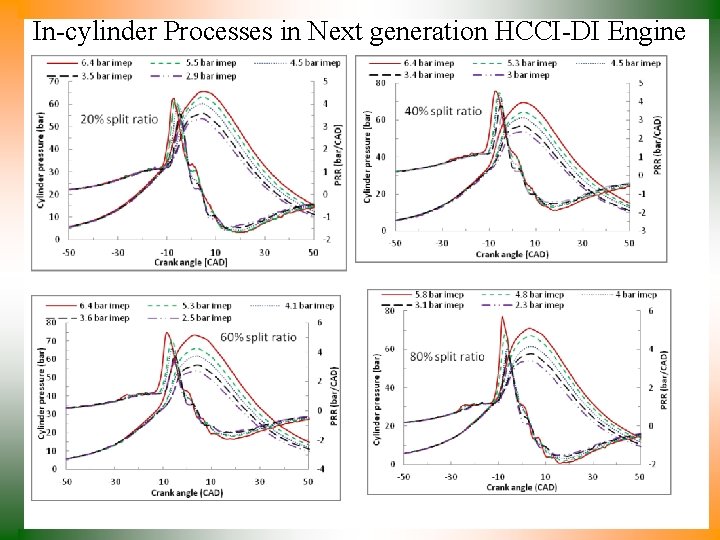
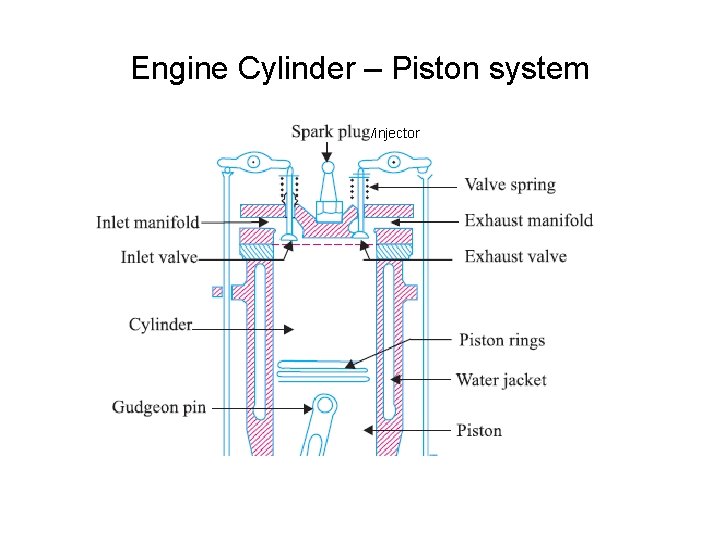
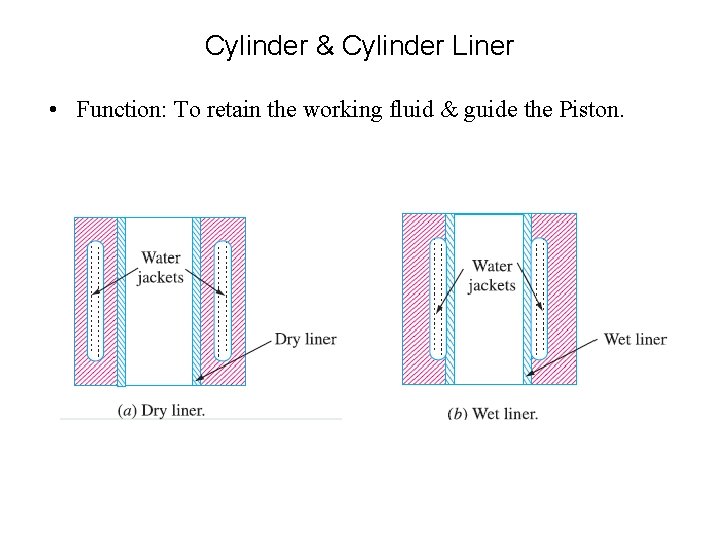
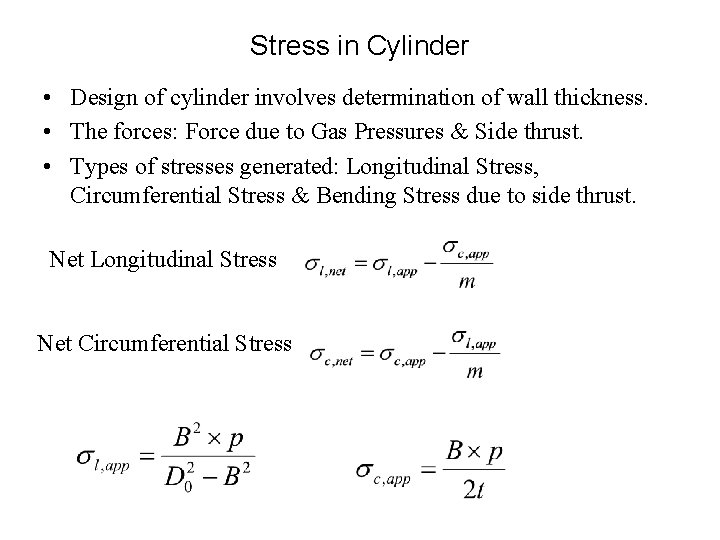
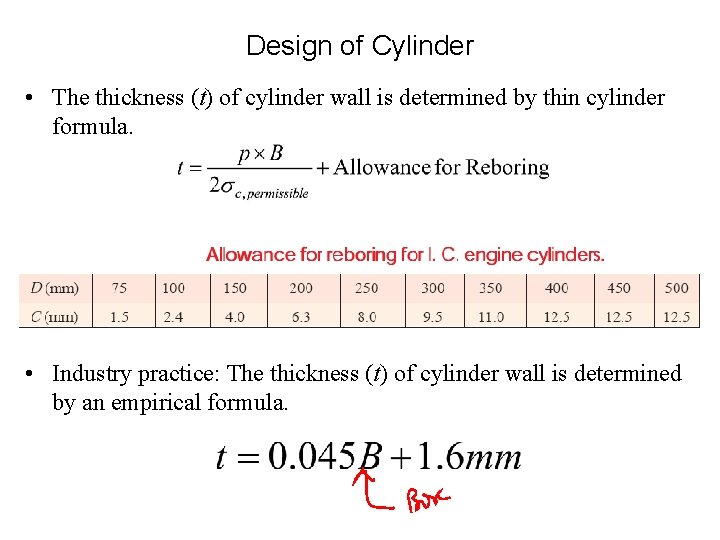
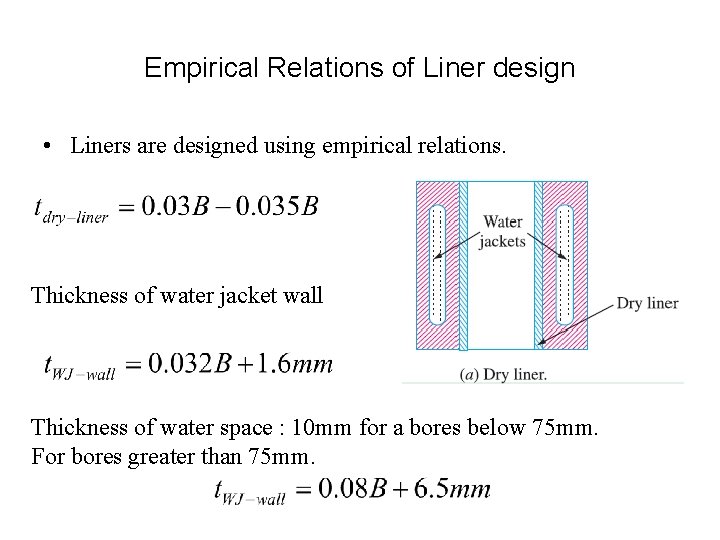
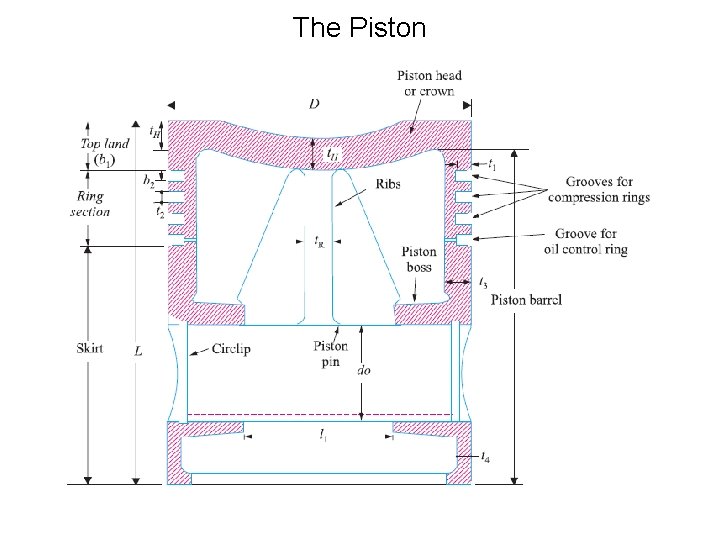
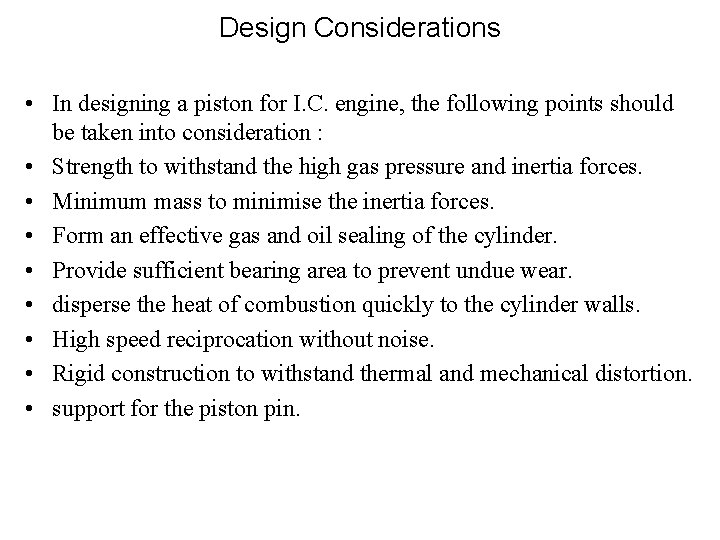
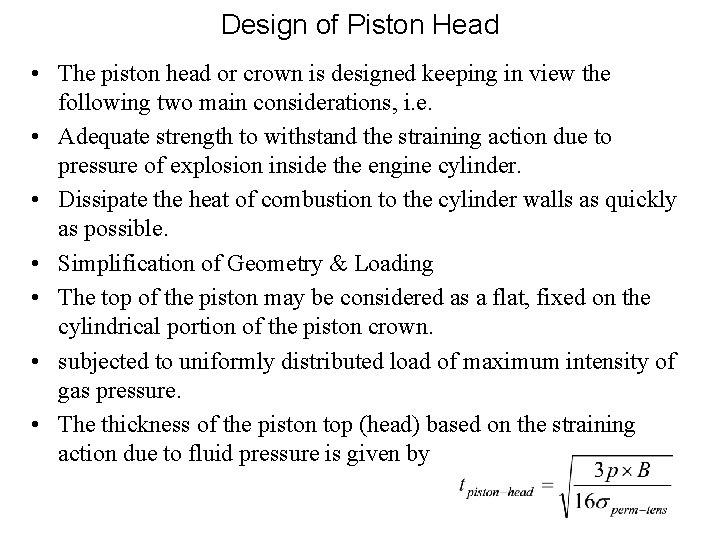
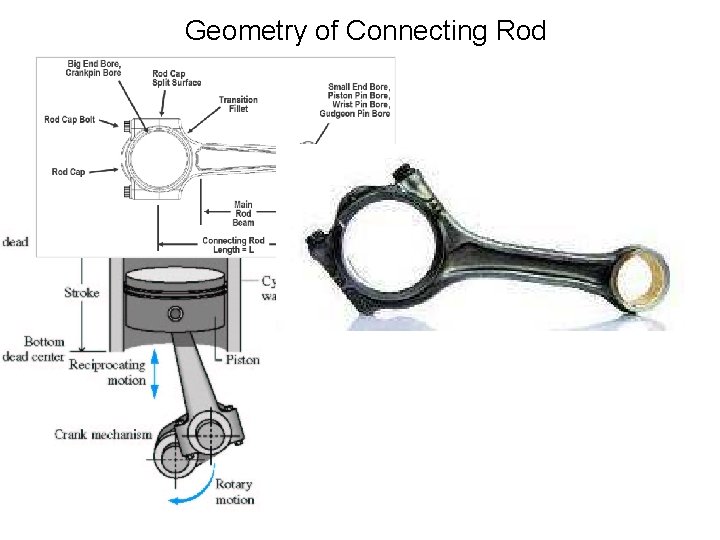
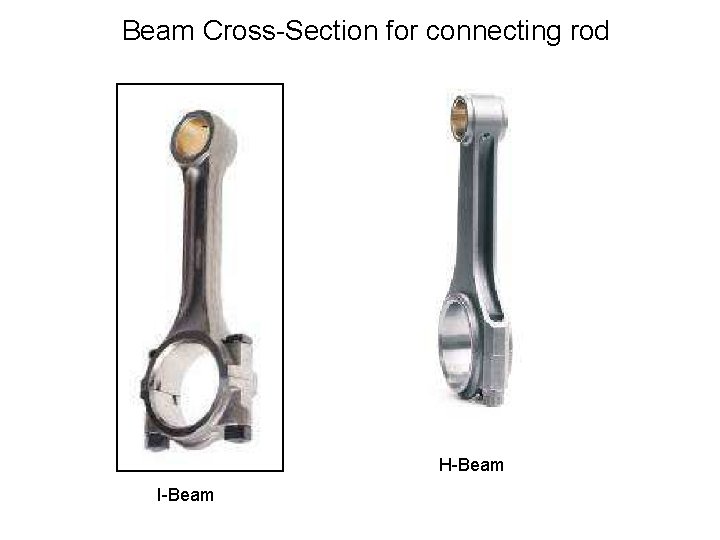
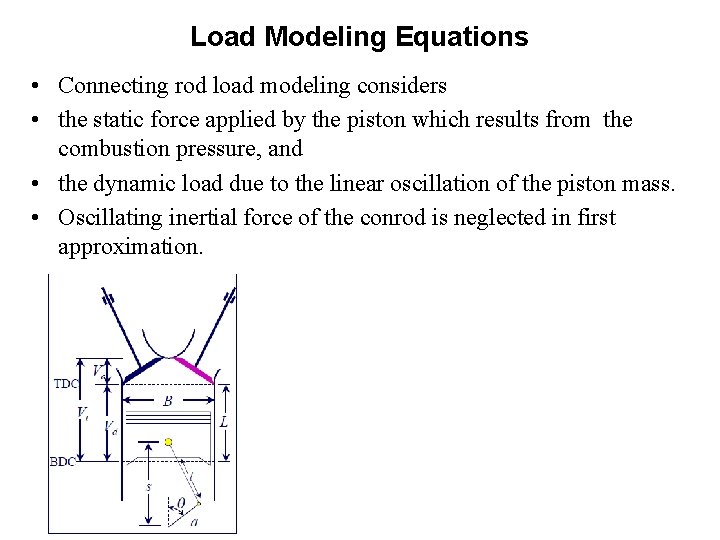
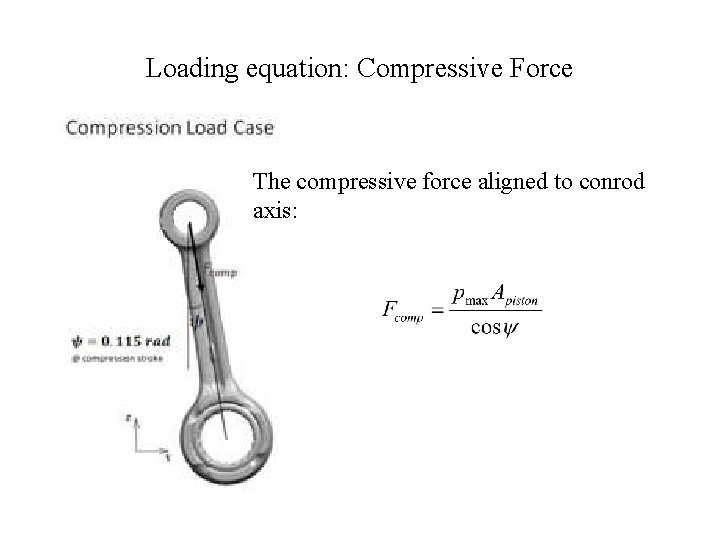
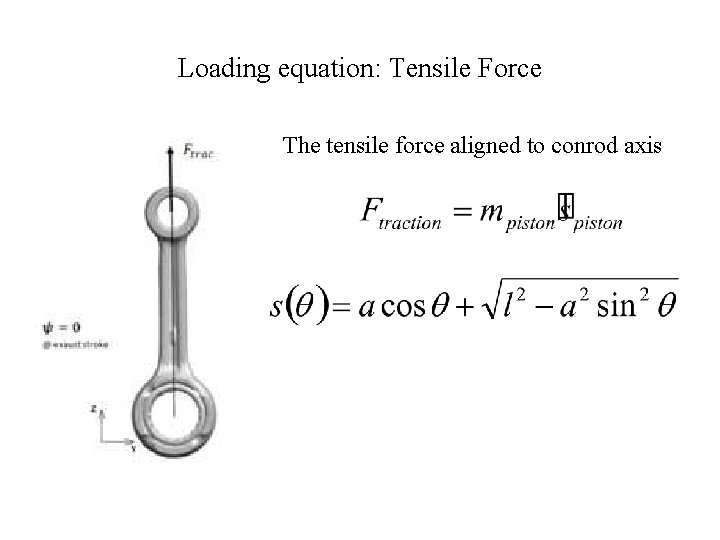
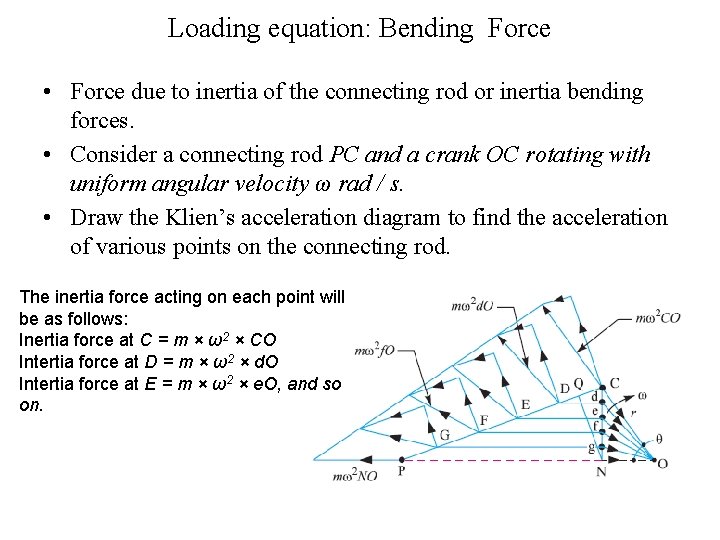
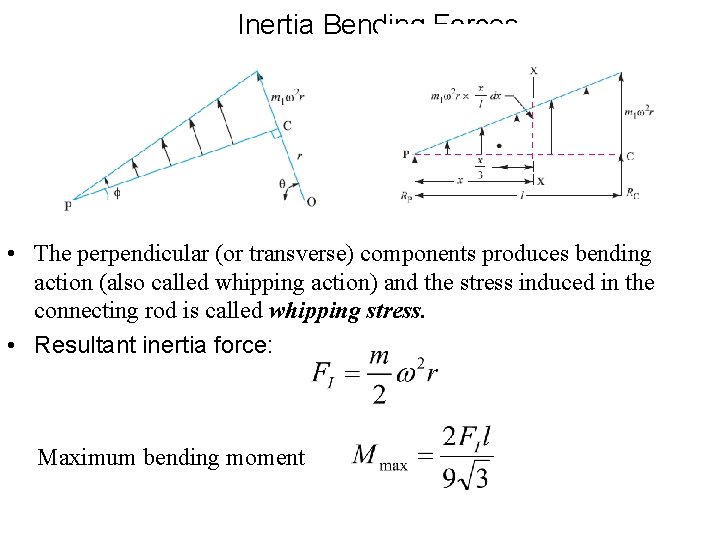
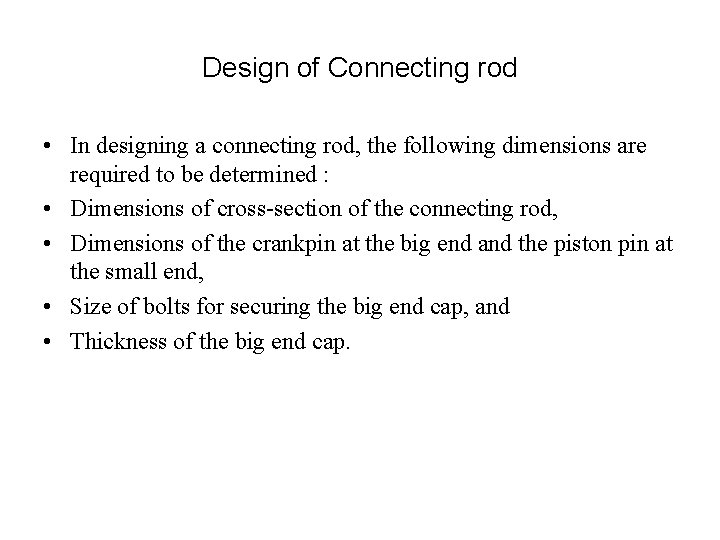
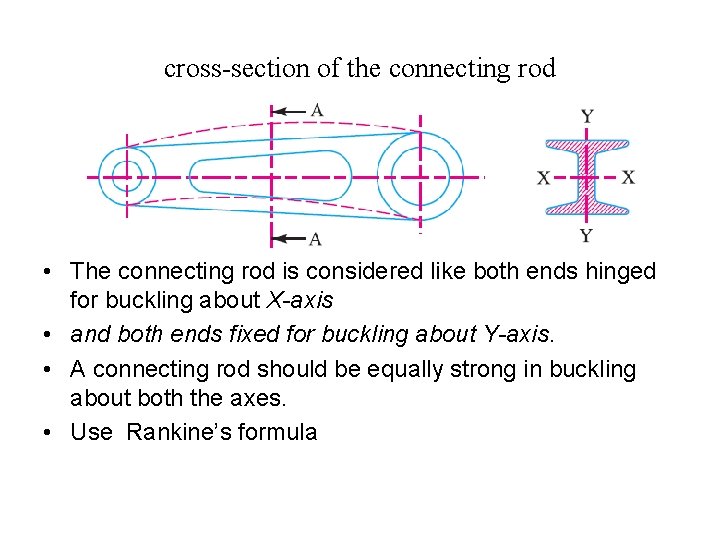
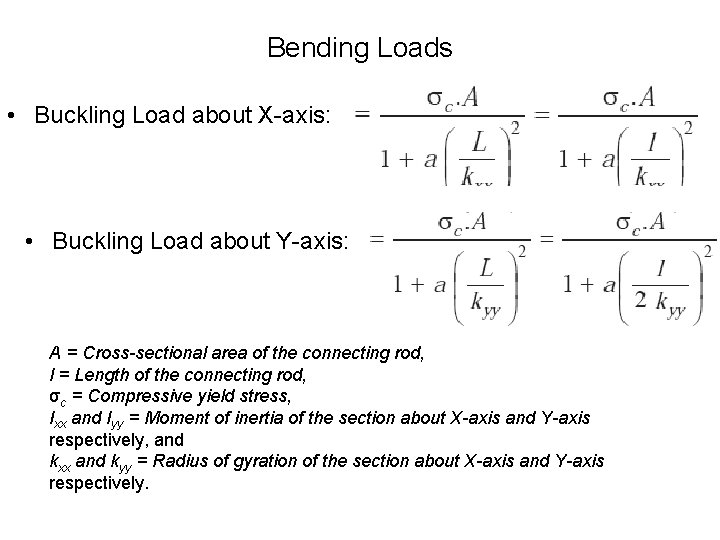
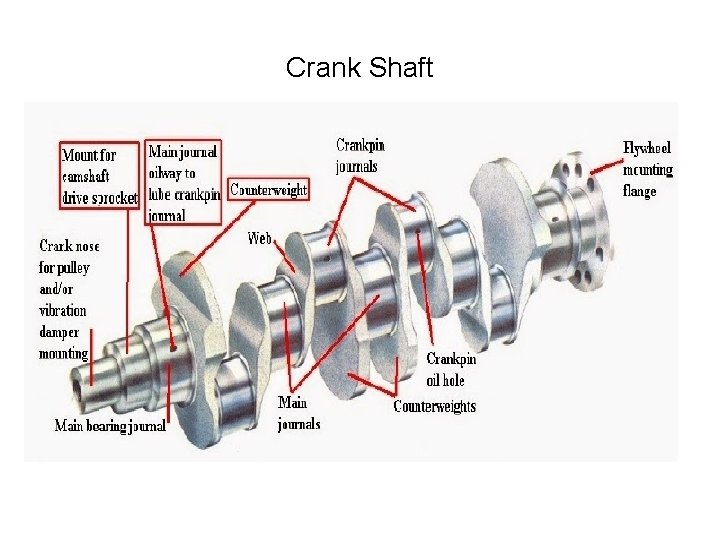
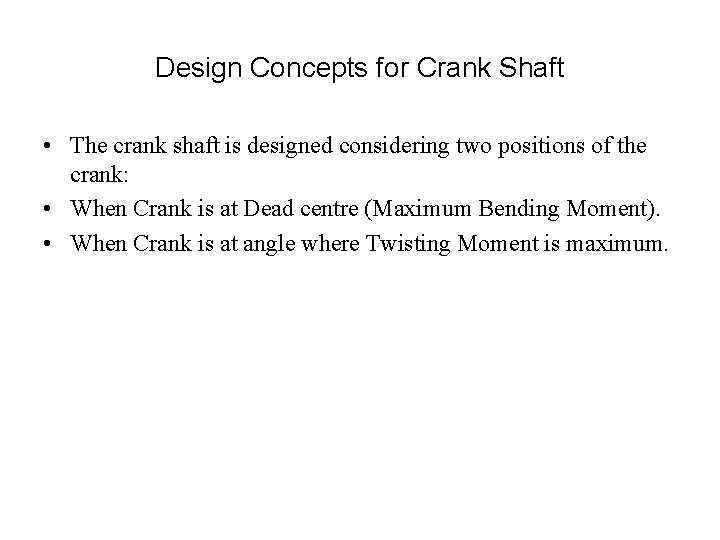
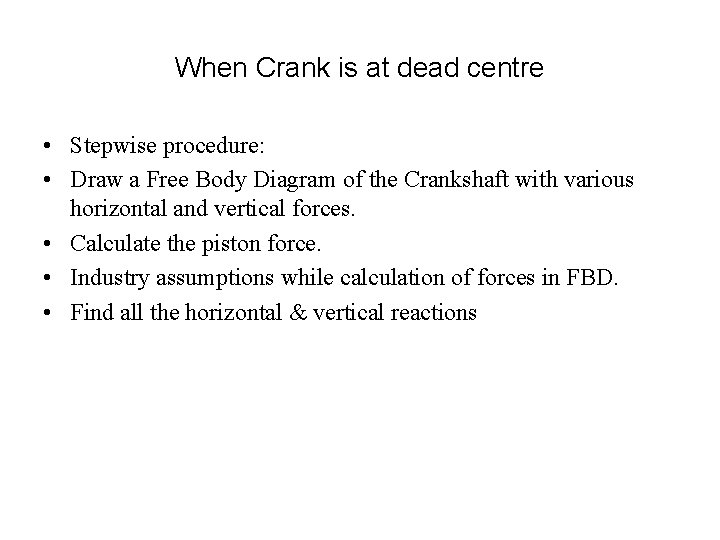
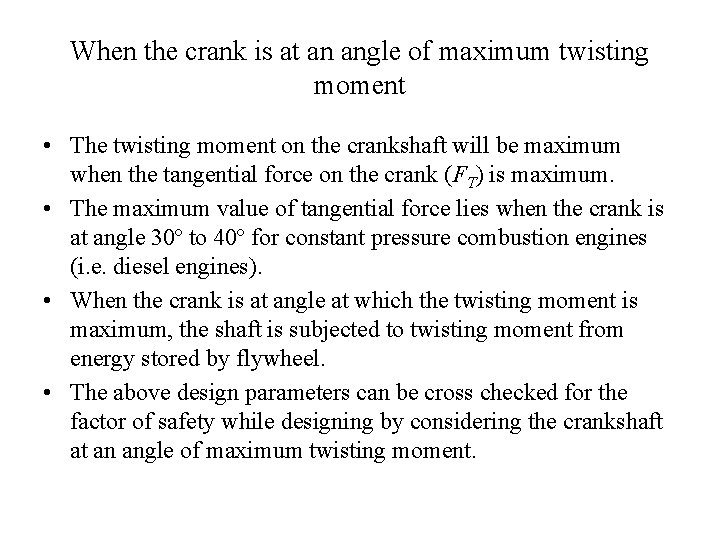
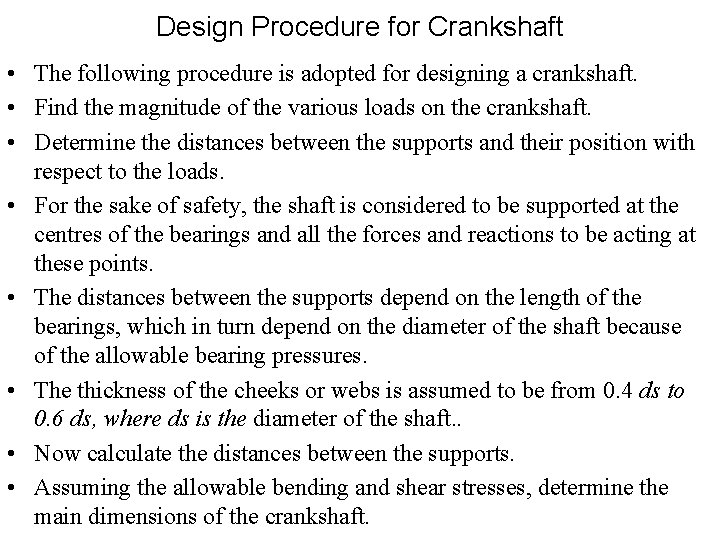
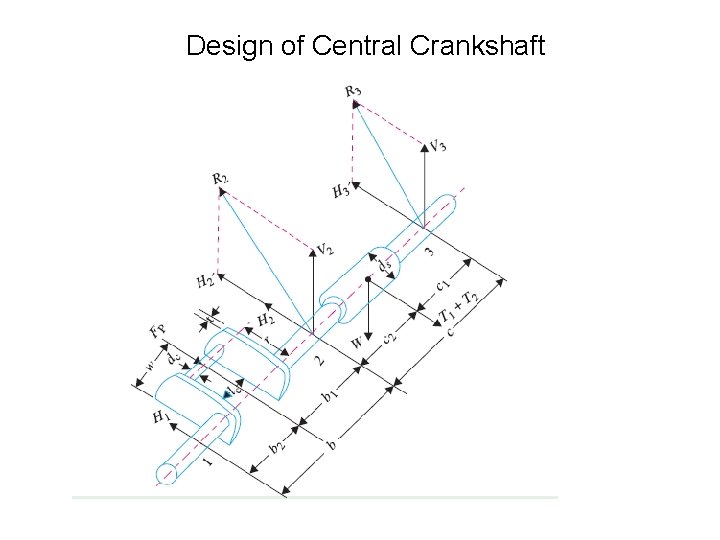
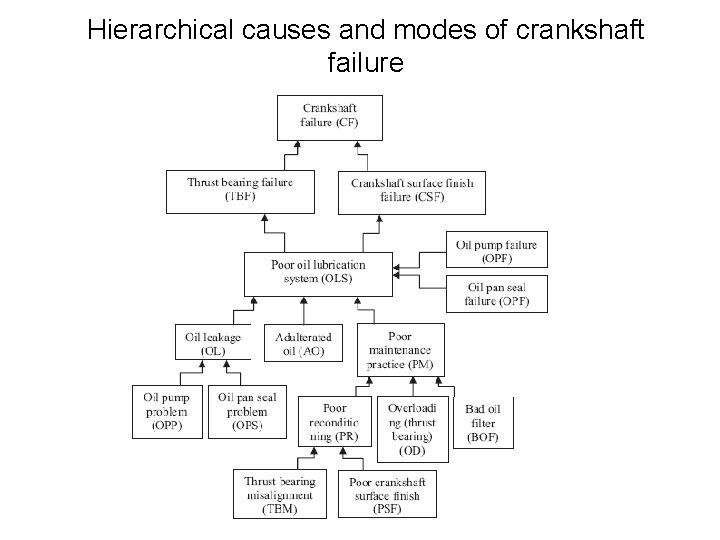
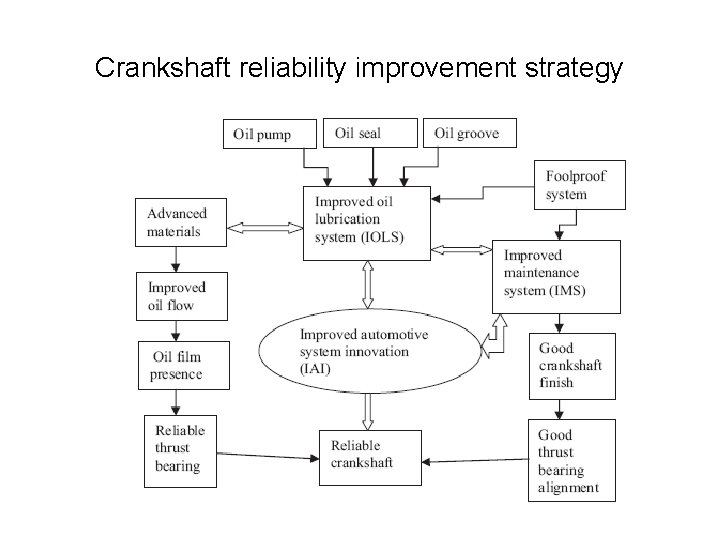
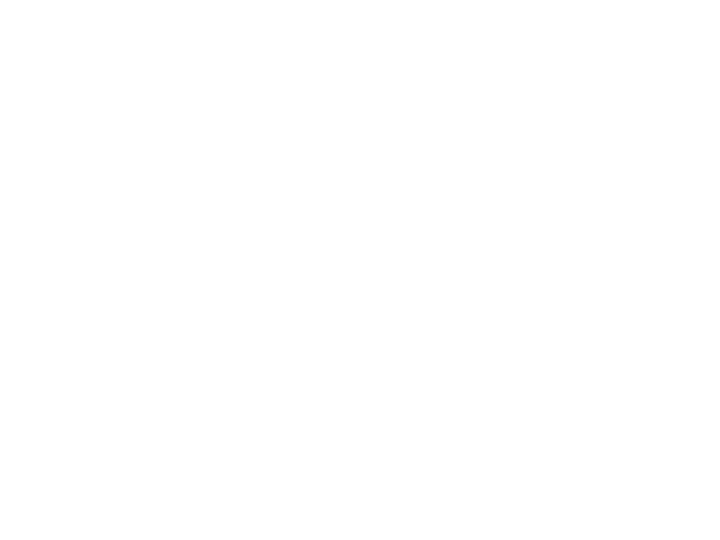
- Slides: 60
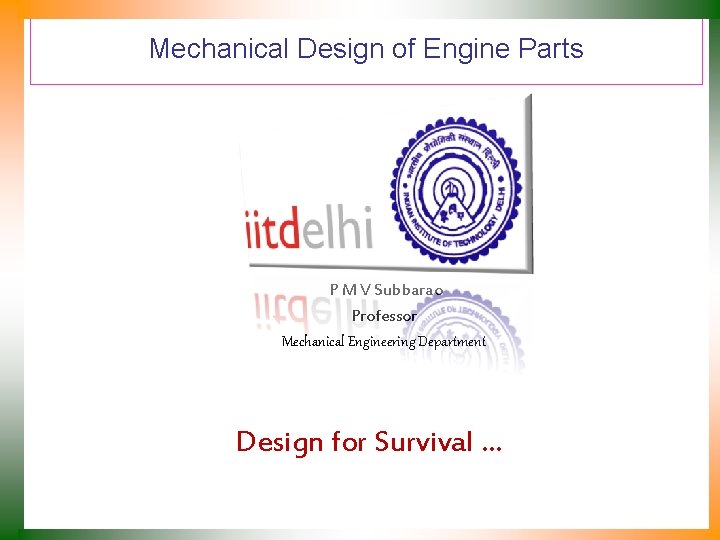
Mechanical Design of Engine Parts P M V Subbarao Professor Mechanical Engineering Department Design for Survival …
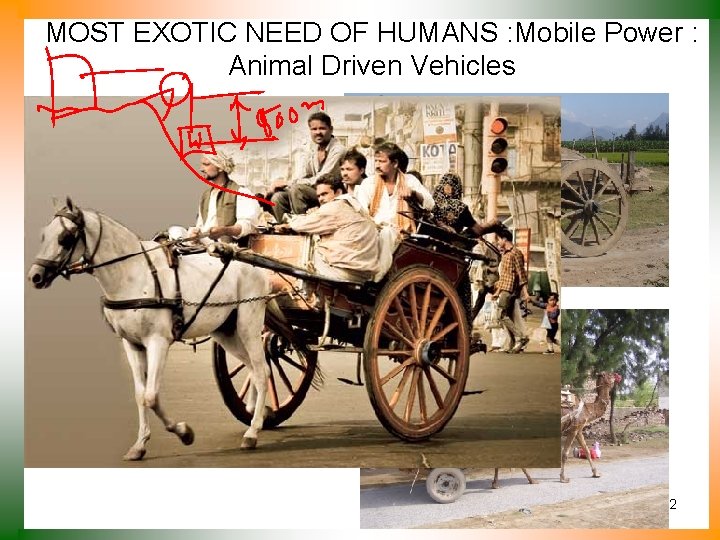
MOST EXOTIC NEED OF HUMANS : Mobile Power : Animal Driven Vehicles 2
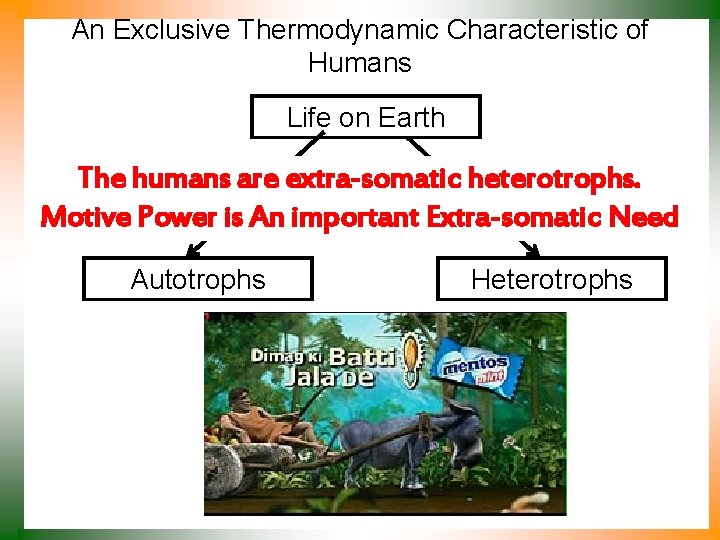
An Exclusive Thermodynamic Characteristic of Humans Life on Earth The humans are extra-somatic heterotrophs. Motive Power is An important Extra-somatic Need Autotrophs Heterotrophs
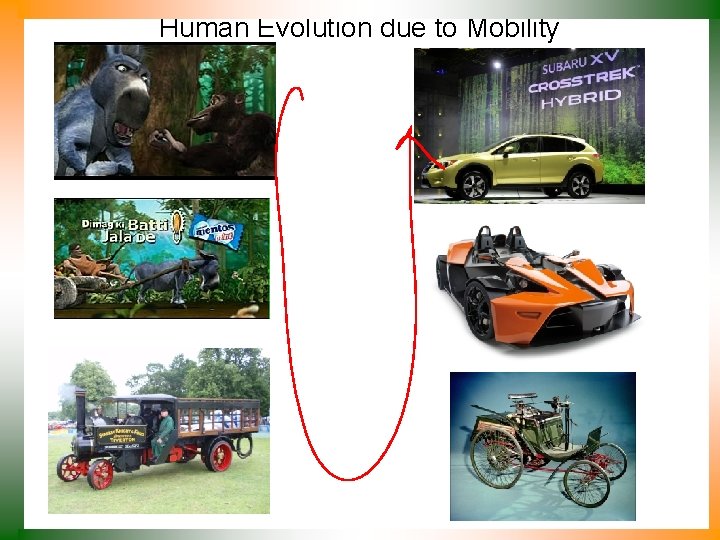
Human Evolution due to Mobility
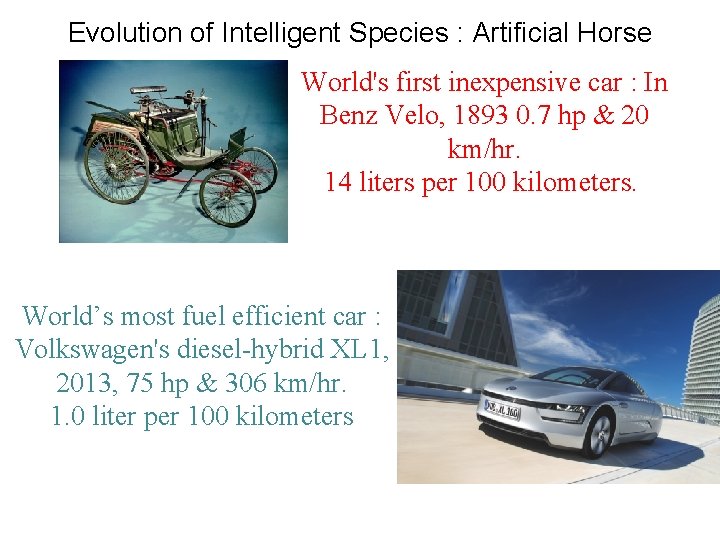
Evolution of Intelligent Species : Artificial Horse World's first inexpensive car : In Benz Velo, 1893 0. 7 hp & 20 km/hr. 14 liters per 100 kilometers. World’s most fuel efficient car : Volkswagen's diesel-hybrid XL 1, 2013, 75 hp & 306 km/hr. 1. 0 liter per 100 kilometers
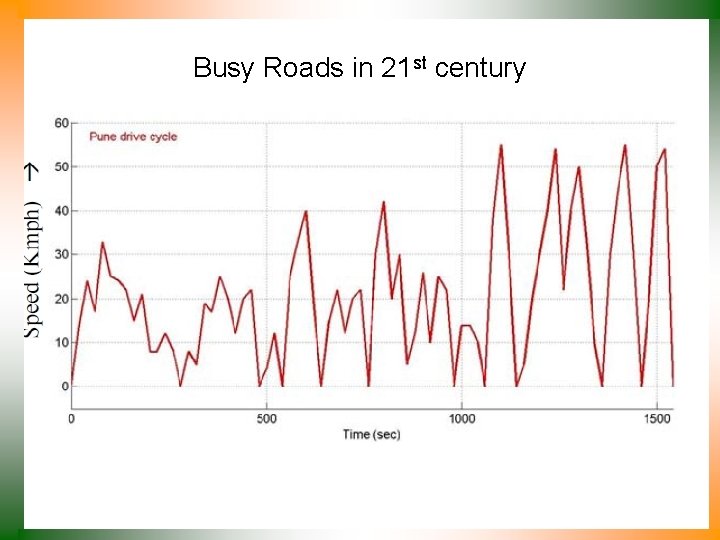
Busy Roads in 21 st century
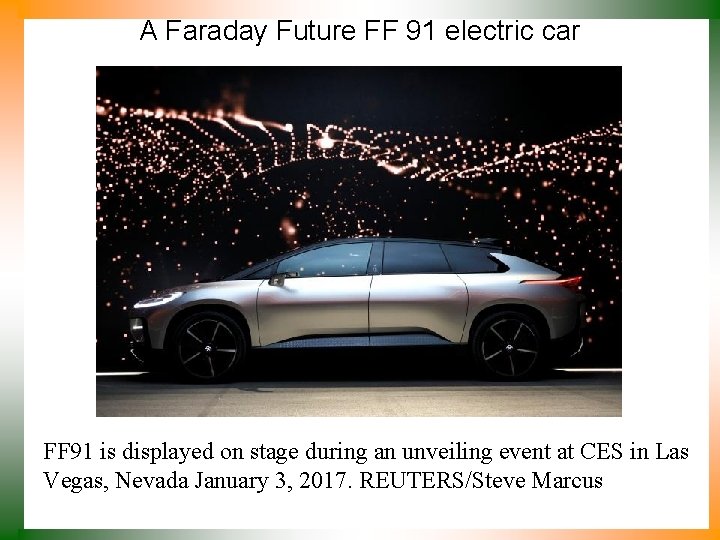
A Faraday Future FF 91 electric car FF 91 is displayed on stage during an unveiling event at CES in Las Vegas, Nevada January 3, 2017. REUTERS/Steve Marcus
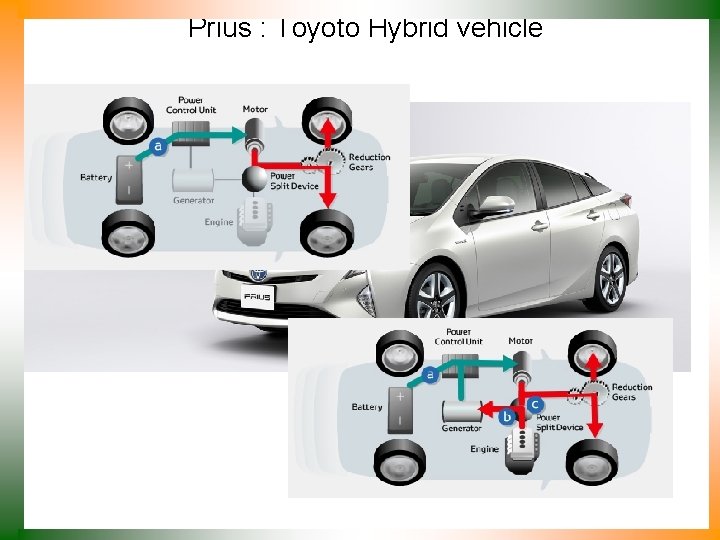
Prius : Toyoto Hybrid vehicle
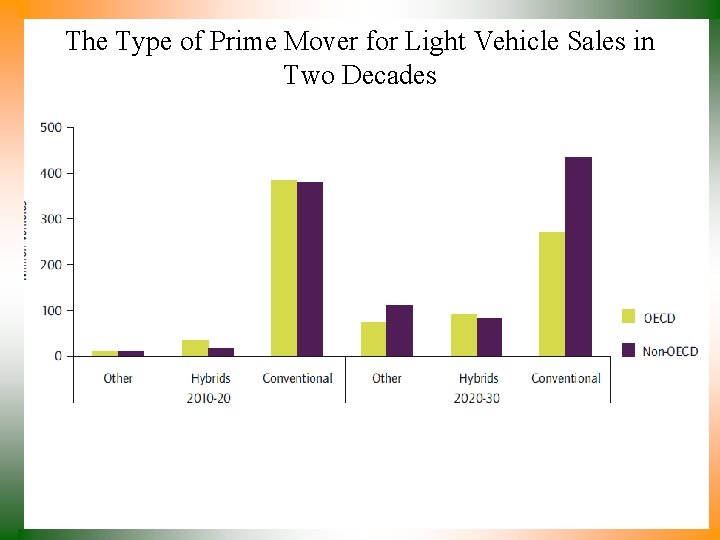
The Type of Prime Mover for Light Vehicle Sales in Two Decades
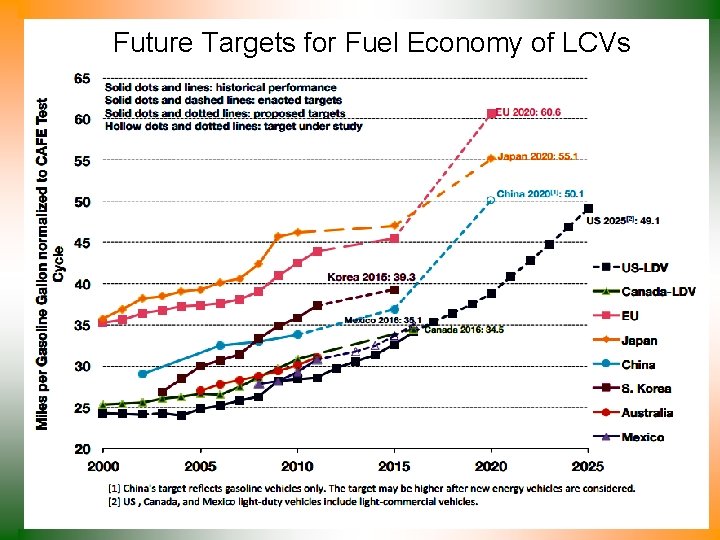
Future Targets for Fuel Economy of LCVs
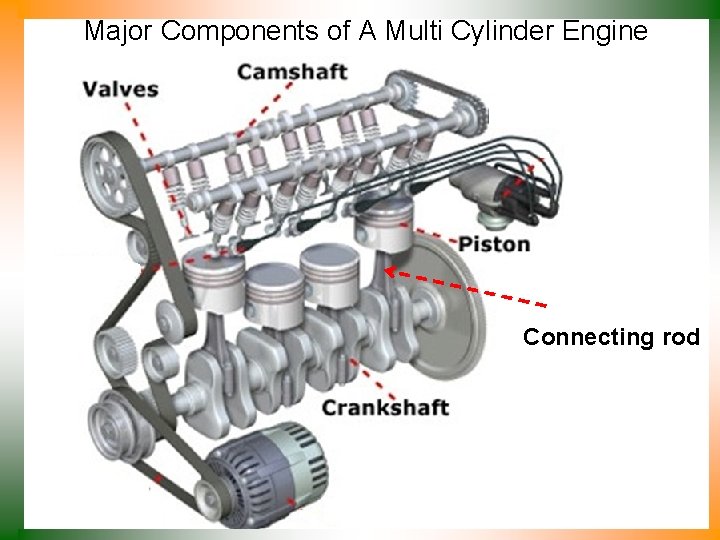
Major Components of A Multi Cylinder Engine Connecting rod
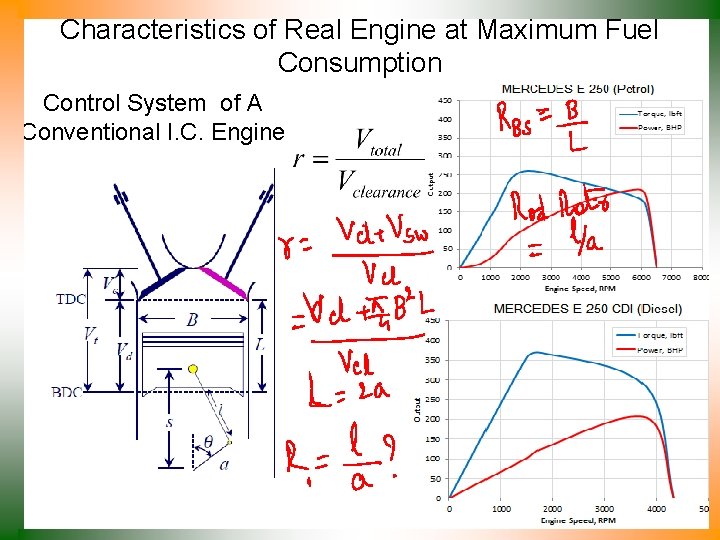
Characteristics of Real Engine at Maximum Fuel Consumption Control System of A Conventional I. C. Engine 12
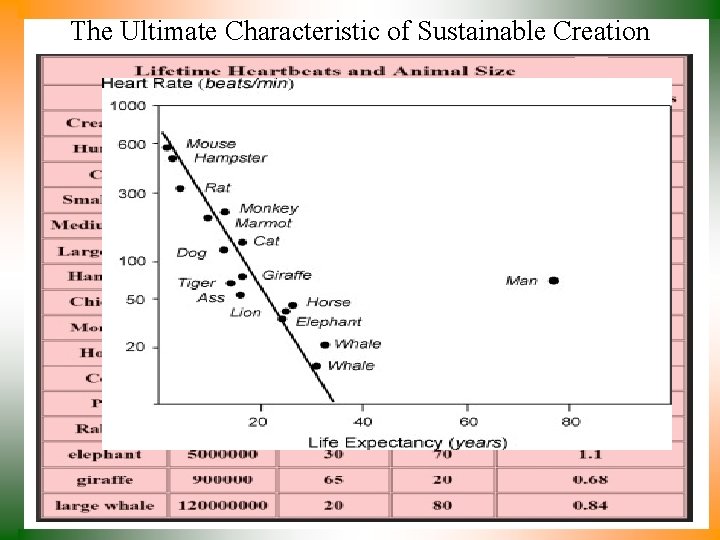
The Ultimate Characteristic of Sustainable Creation
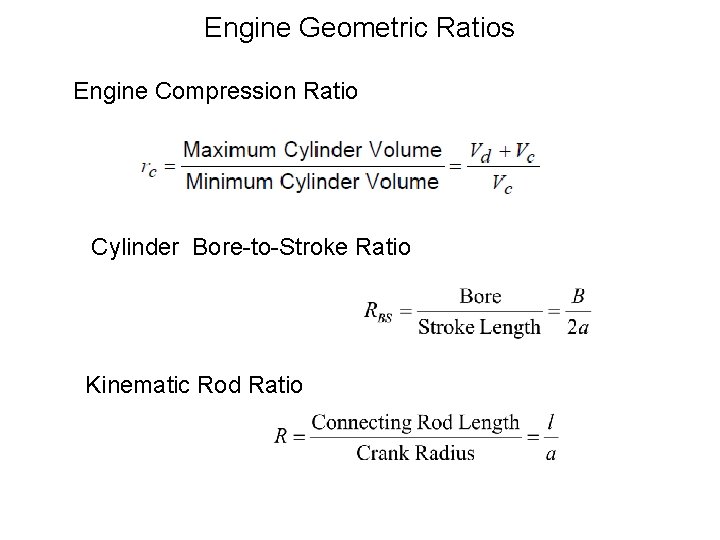
Engine Geometric Ratios Engine Compression Ratio Cylinder Bore-to-Stroke Ratio Kinematic Rod Ratio
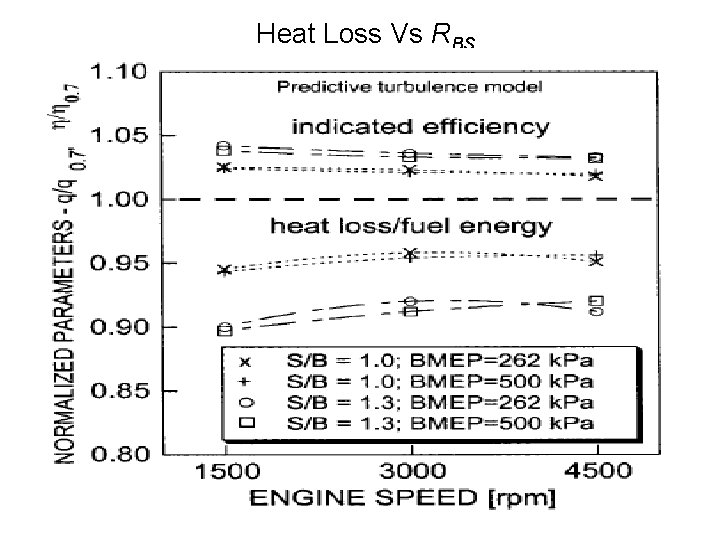
Heat Loss Vs RBS
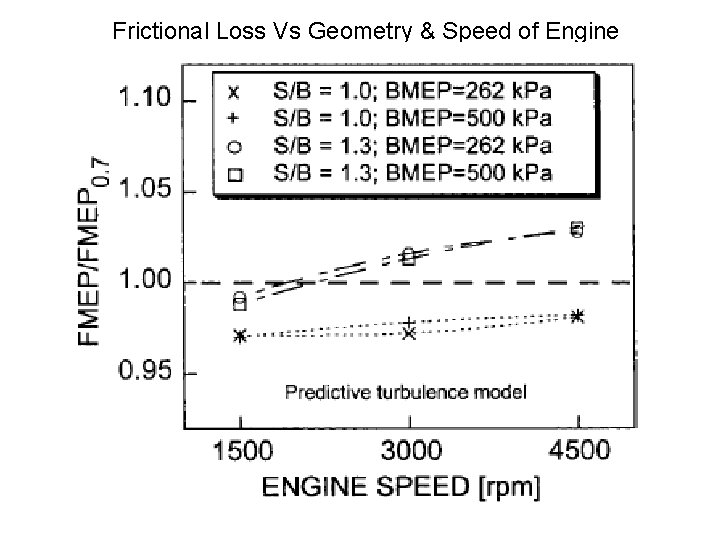
Frictional Loss Vs Geometry & Speed of Engine
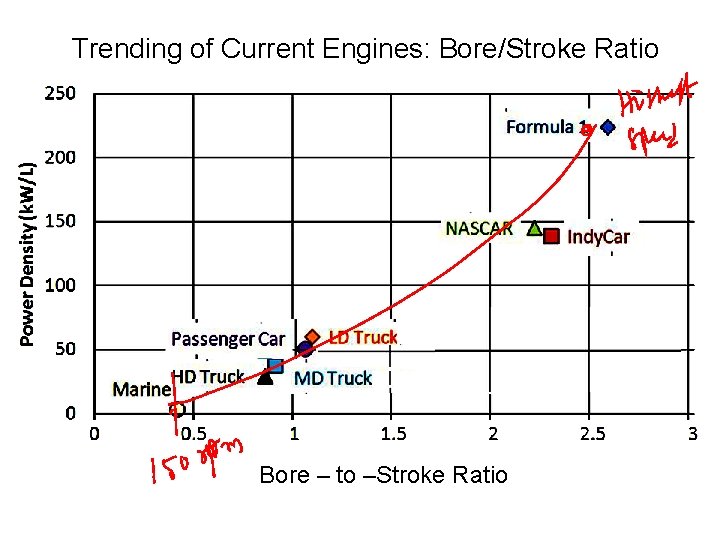
Trending of Current Engines: Bore/Stroke Ratio Bore – to –Stroke Ratio
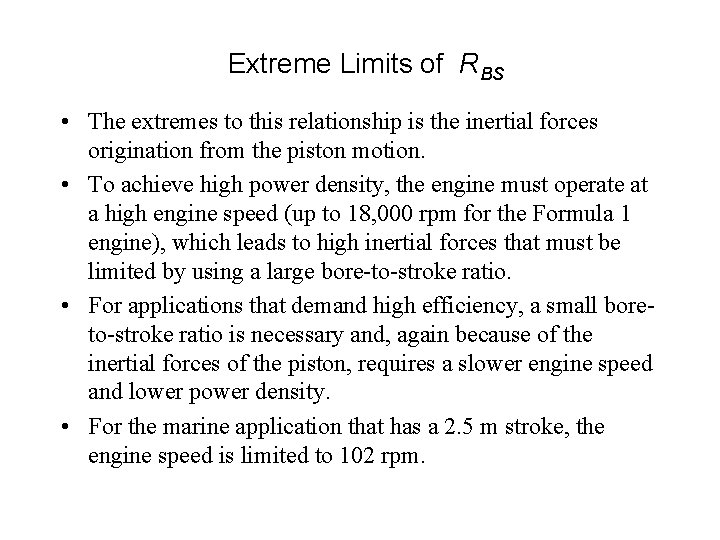
Extreme Limits of RBS • The extremes to this relationship is the inertial forces origination from the piston motion. • To achieve high power density, the engine must operate at a high engine speed (up to 18, 000 rpm for the Formula 1 engine), which leads to high inertial forces that must be limited by using a large bore-to-stroke ratio. • For applications that demand high efficiency, a small boreto-stroke ratio is necessary and, again because of the inertial forces of the piston, requires a slower engine speed and lower power density. • For the marine application that has a 2. 5 m stroke, the engine speed is limited to 102 rpm.
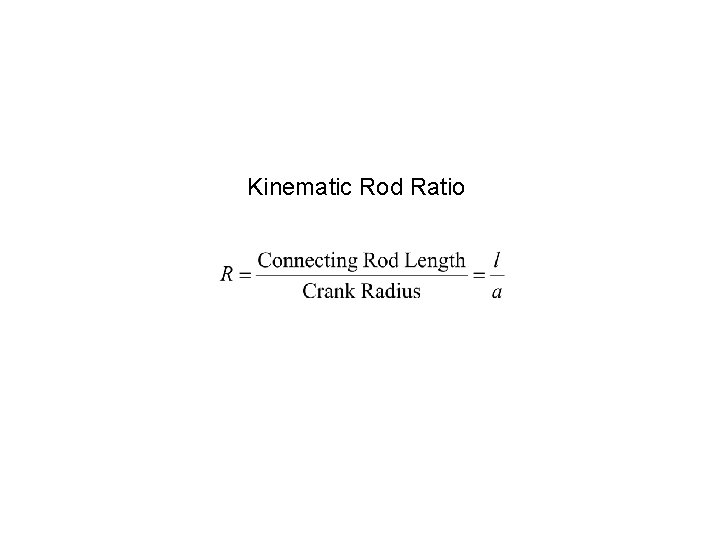
Kinematic Rod Ratio
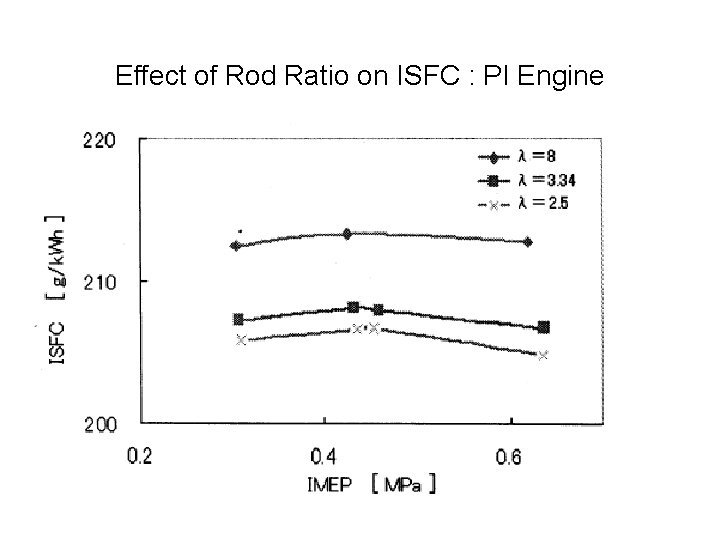
Effect of Rod Ratio on ISFC : PI Engine
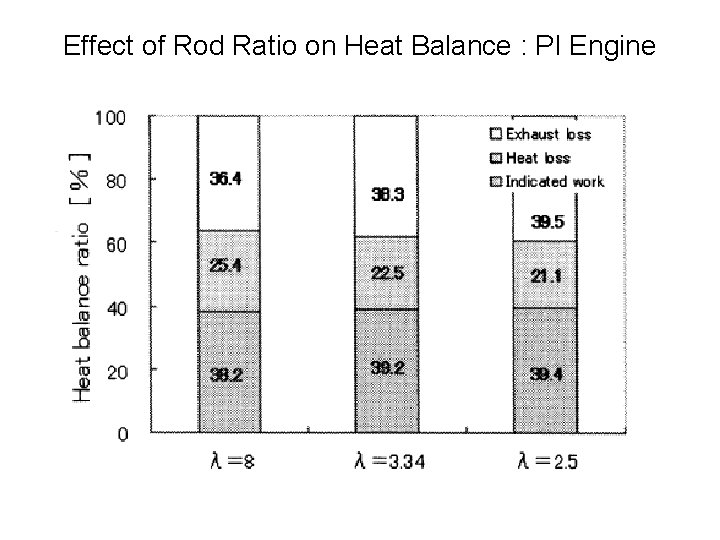
Effect of Rod Ratio on Heat Balance : PI Engine
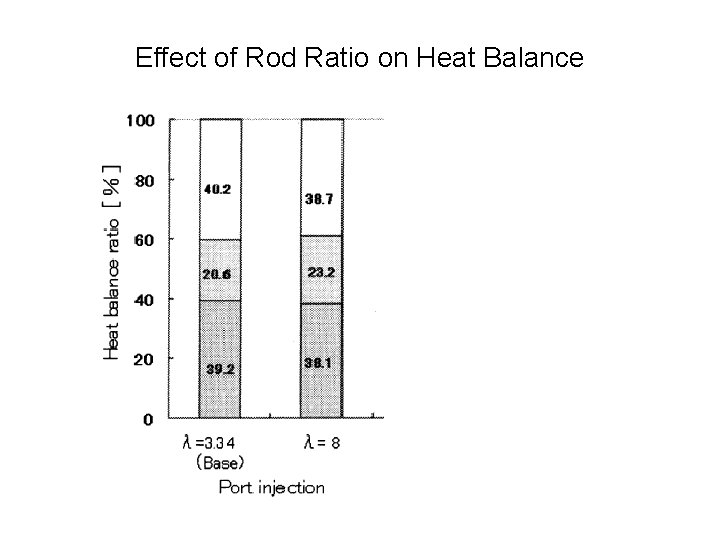
Effect of Rod Ratio on Heat Balance
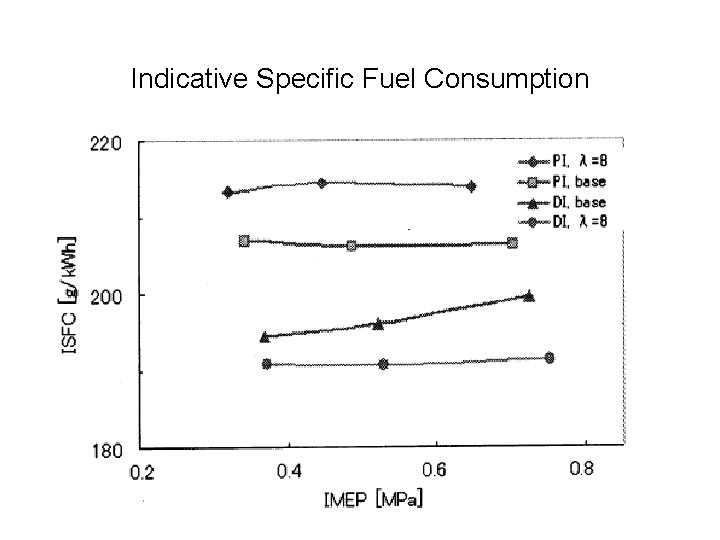
Indicative Specific Fuel Consumption
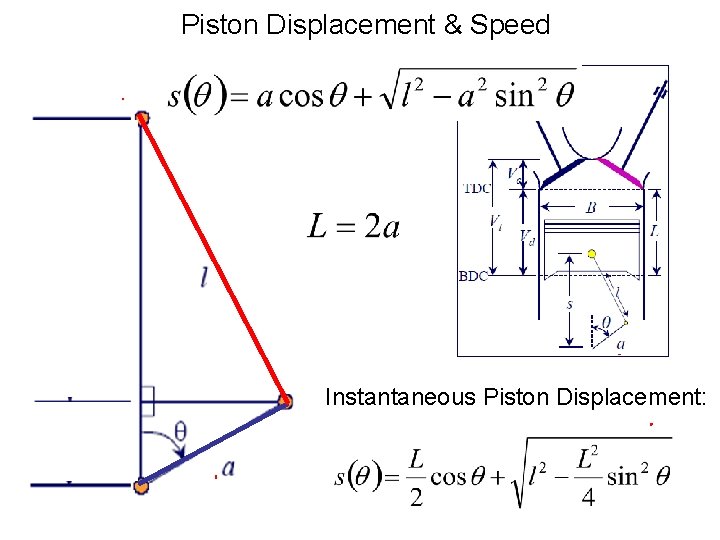
Piston Displacement & Speed Instantaneous Piston Displacement:
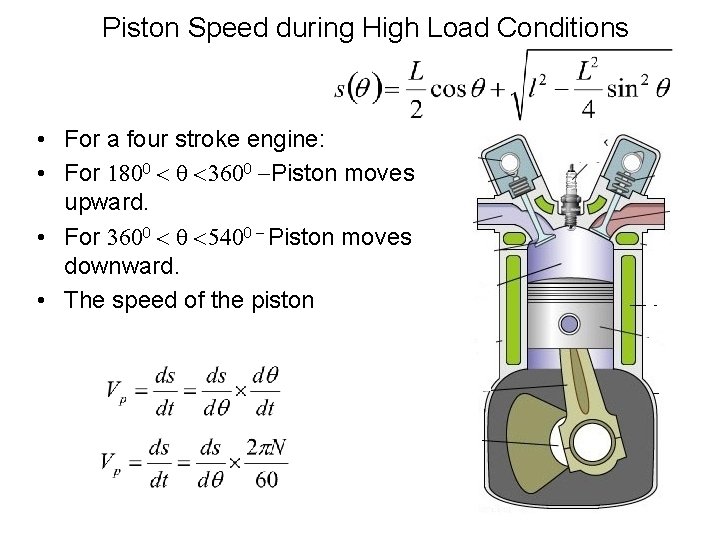
Piston Speed during High Load Conditions • For a four stroke engine: • For 1800 < q <3600 -Piston moves upward. • For 3600 < q <5400 - Piston moves downward. • The speed of the piston
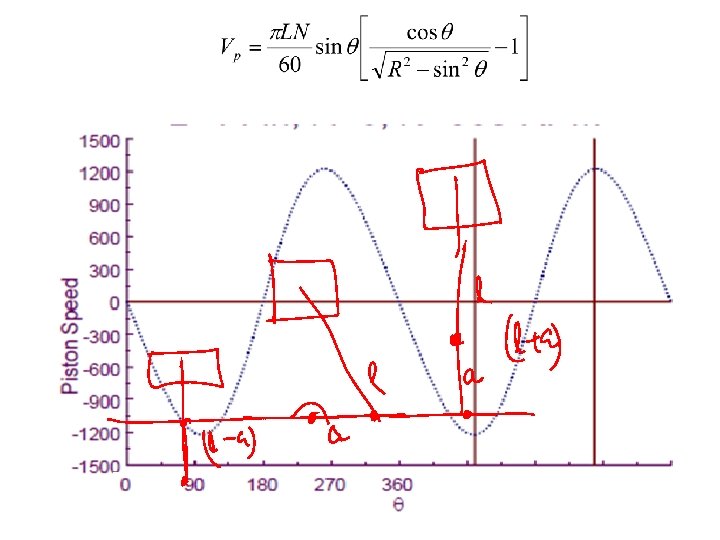
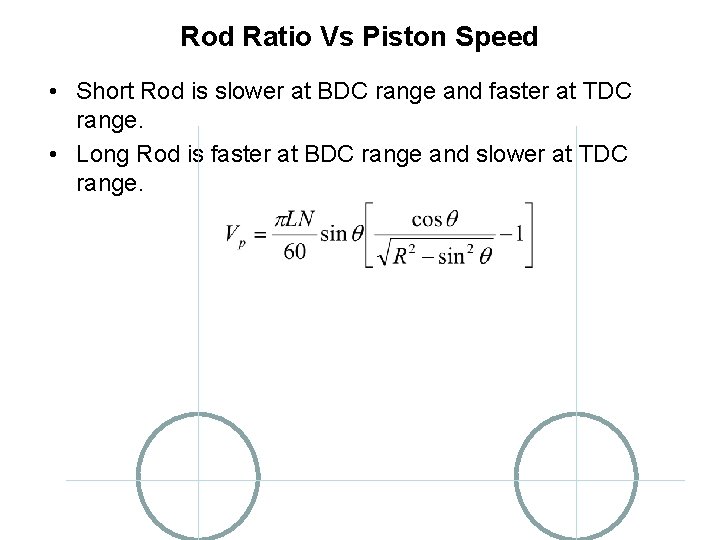
Rod Ratio Vs Piston Speed • Short Rod is slower at BDC range and faster at TDC range. • Long Rod is faster at BDC range and slower at TDC range.
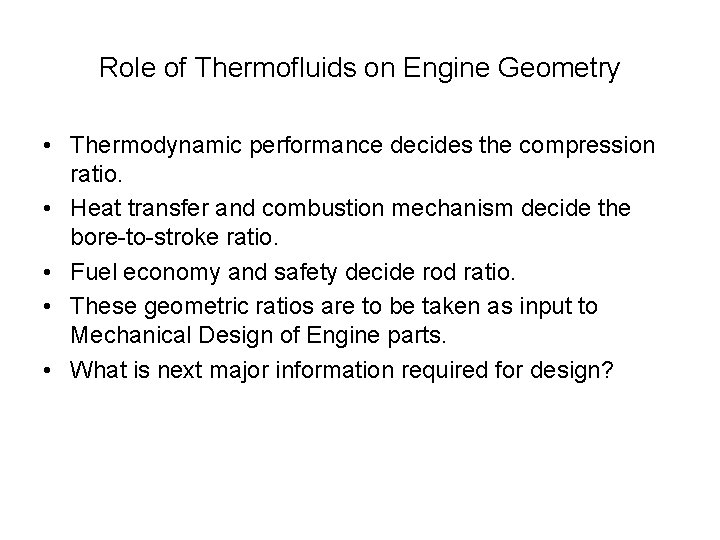
Role of Thermofluids on Engine Geometry • Thermodynamic performance decides the compression ratio. • Heat transfer and combustion mechanism decide the bore-to-stroke ratio. • Fuel economy and safety decide rod ratio. • These geometric ratios are to be taken as input to Mechanical Design of Engine parts. • What is next major information required for design?
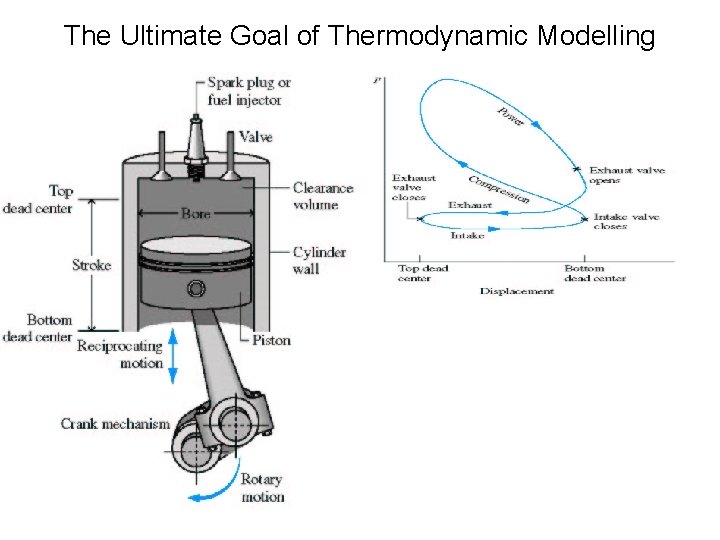
The Ultimate Goal of Thermodynamic Modelling
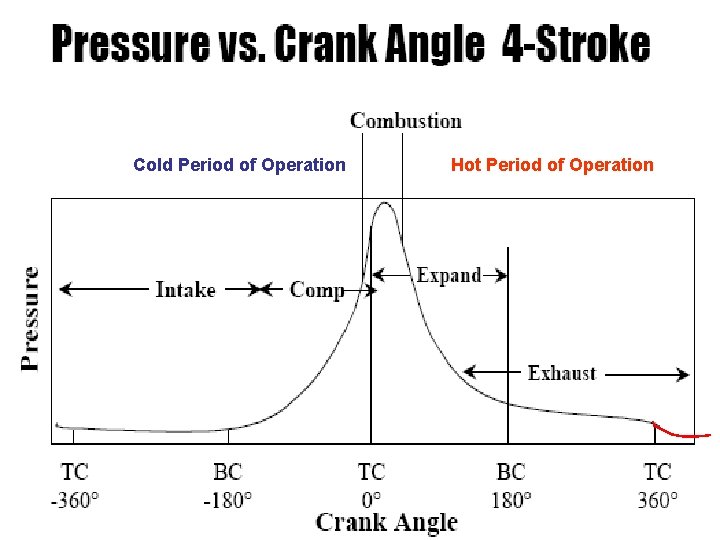
Cold Period of Operation Hot Period of Operation
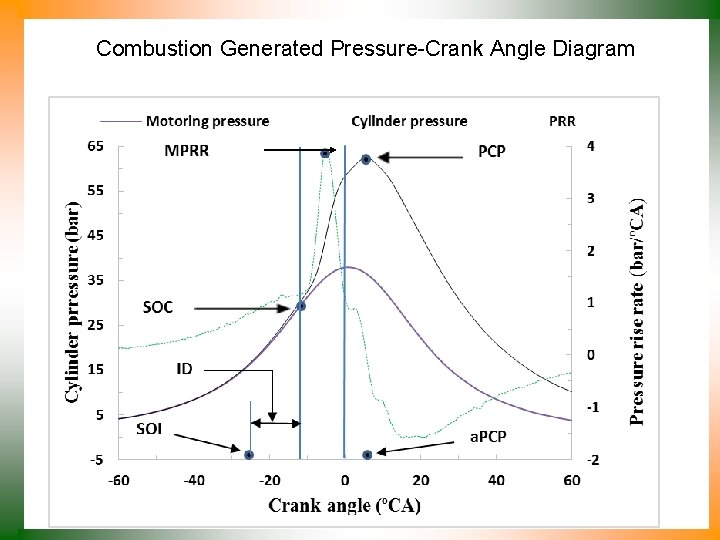
Combustion Generated Pressure-Crank Angle Diagram
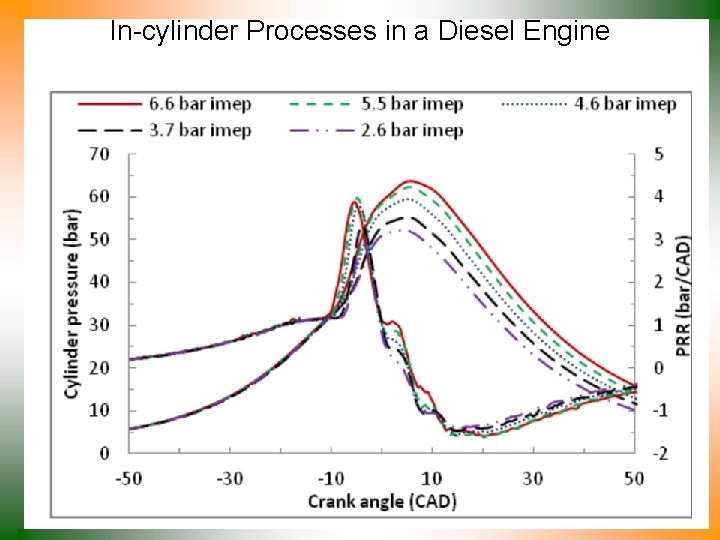
In-cylinder Processes in a Diesel Engine
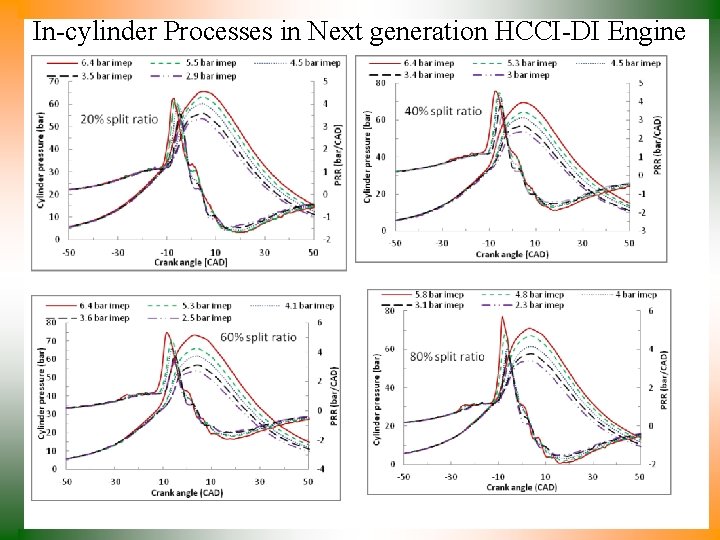
In-cylinder Processes in Next generation HCCI-DI Engine
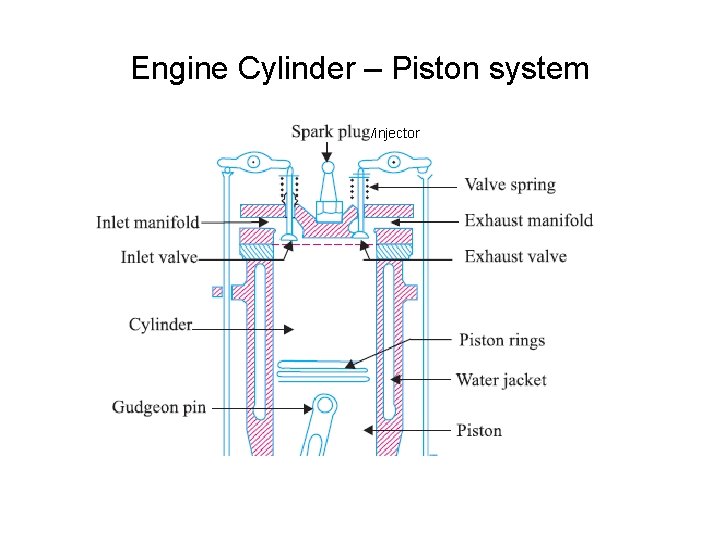
Engine Cylinder – Piston system /injector
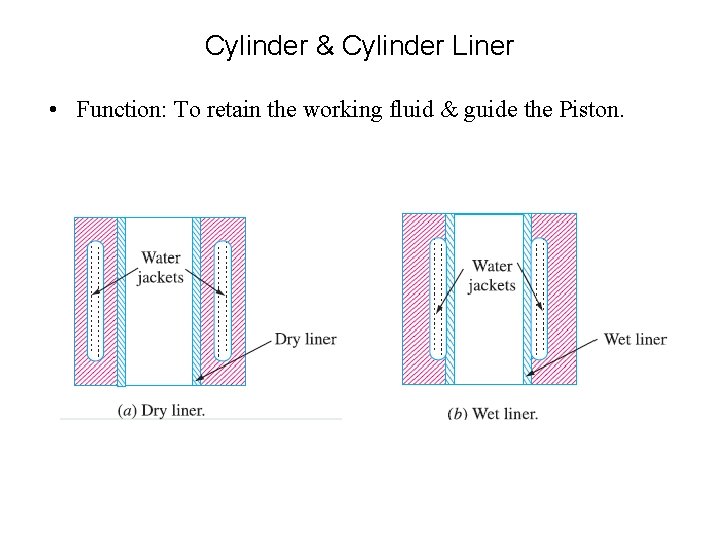
Cylinder & Cylinder Liner • Function: To retain the working fluid & guide the Piston.
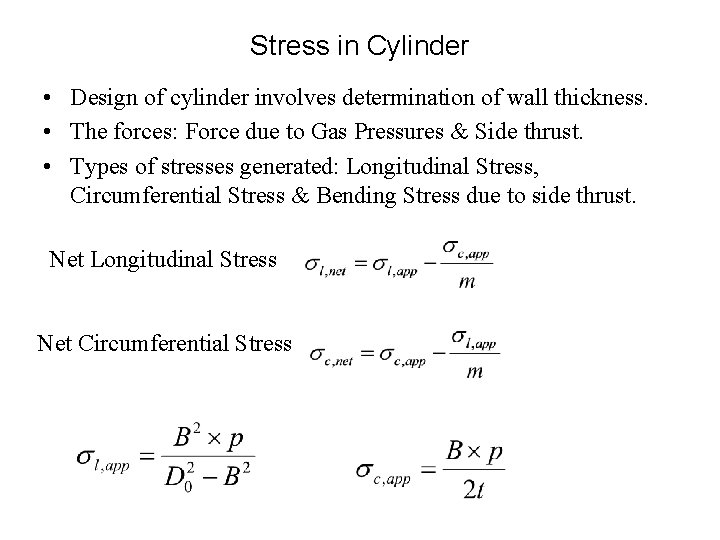
Stress in Cylinder • Design of cylinder involves determination of wall thickness. • The forces: Force due to Gas Pressures & Side thrust. • Types of stresses generated: Longitudinal Stress, Circumferential Stress & Bending Stress due to side thrust. Net Longitudinal Stress Net Circumferential Stress
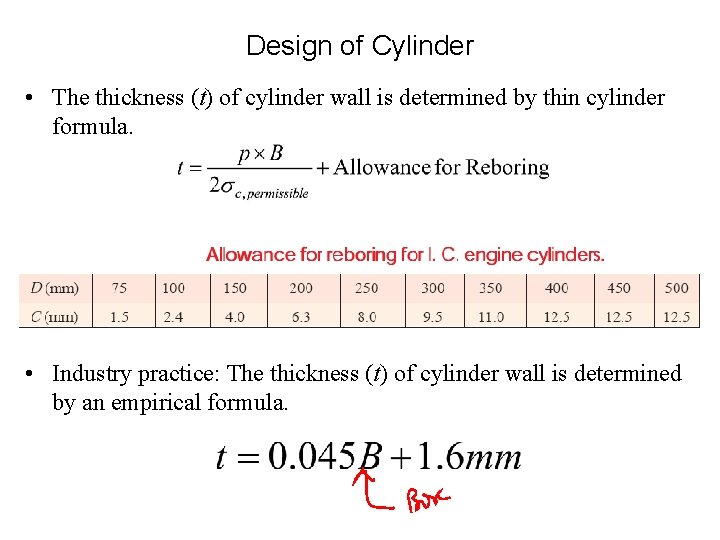
Design of Cylinder • The thickness (t) of cylinder wall is determined by thin cylinder formula. • Industry practice: The thickness (t) of cylinder wall is determined by an empirical formula.
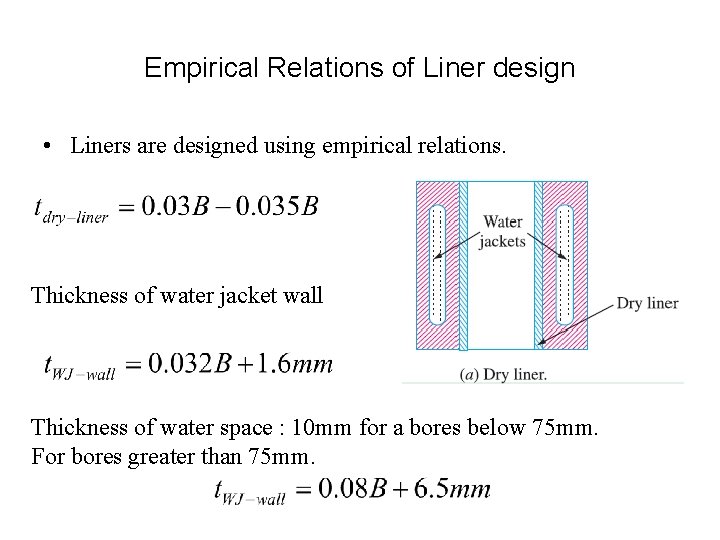
Empirical Relations of Liner design • Liners are designed using empirical relations. Thickness of water jacket wall Thickness of water space : 10 mm for a bores below 75 mm. For bores greater than 75 mm.
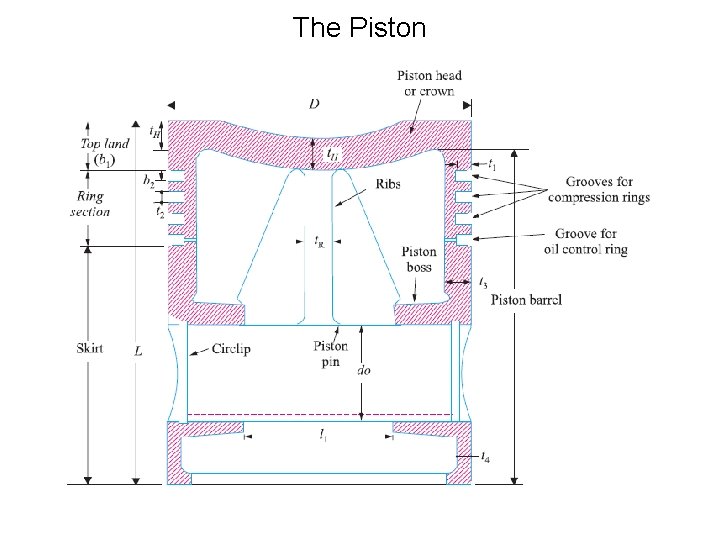
The Piston
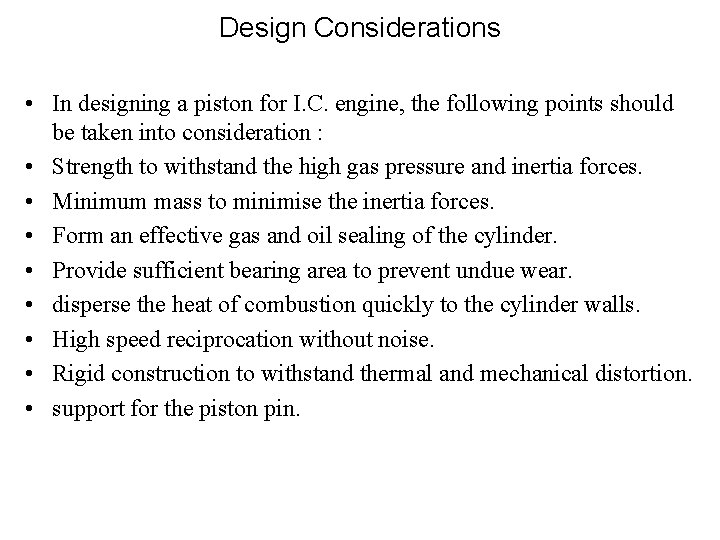
Design Considerations • In designing a piston for I. C. engine, the following points should be taken into consideration : • Strength to withstand the high gas pressure and inertia forces. • Minimum mass to minimise the inertia forces. • Form an effective gas and oil sealing of the cylinder. • Provide sufficient bearing area to prevent undue wear. • disperse the heat of combustion quickly to the cylinder walls. • High speed reciprocation without noise. • Rigid construction to withstand thermal and mechanical distortion. • support for the piston pin.
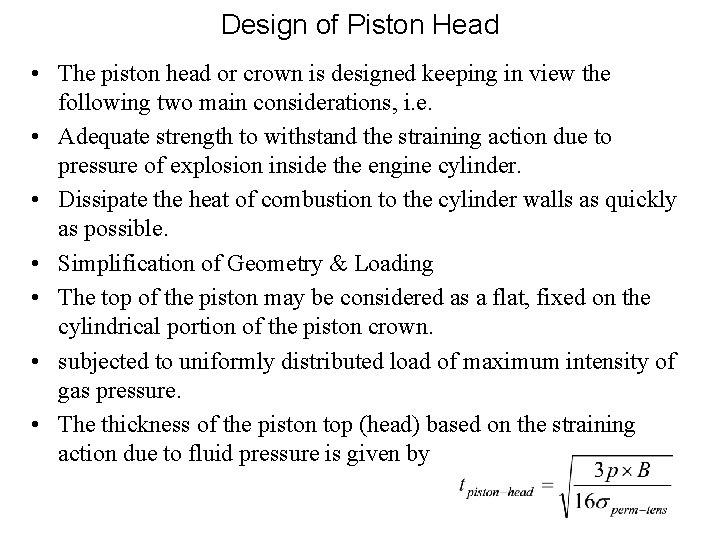
Design of Piston Head • The piston head or crown is designed keeping in view the following two main considerations, i. e. • Adequate strength to withstand the straining action due to pressure of explosion inside the engine cylinder. • Dissipate the heat of combustion to the cylinder walls as quickly as possible. • Simplification of Geometry & Loading • The top of the piston may be considered as a flat, fixed on the cylindrical portion of the piston crown. • subjected to uniformly distributed load of maximum intensity of gas pressure. • The thickness of the piston top (head) based on the straining action due to fluid pressure is given by
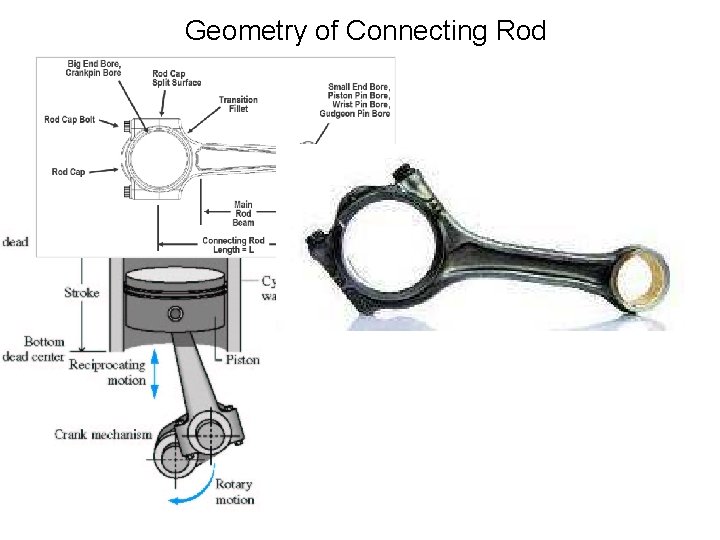
Geometry of Connecting Rod
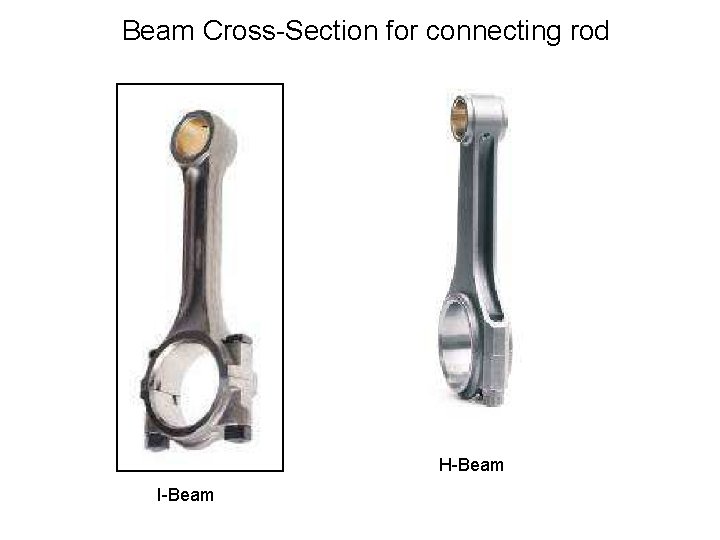
Beam Cross-Section for connecting rod H-Beam I-Beam
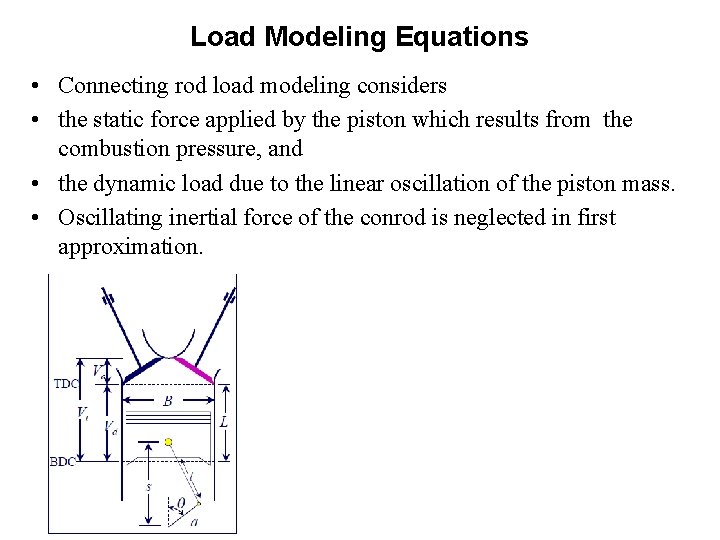
Load Modeling Equations • Connecting rod load modeling considers • the static force applied by the piston which results from the combustion pressure, and • the dynamic load due to the linear oscillation of the piston mass. • Oscillating inertial force of the conrod is neglected in first approximation.
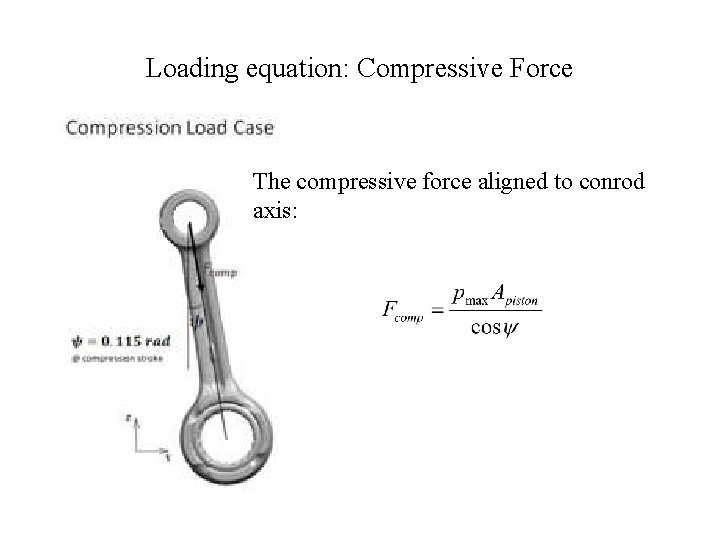
Loading equation: Compressive Force The compressive force aligned to conrod axis:
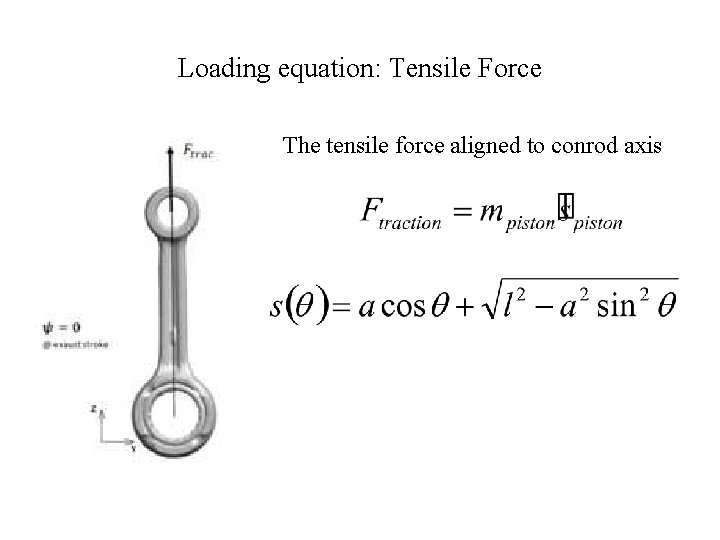
Loading equation: Tensile Force The tensile force aligned to conrod axis
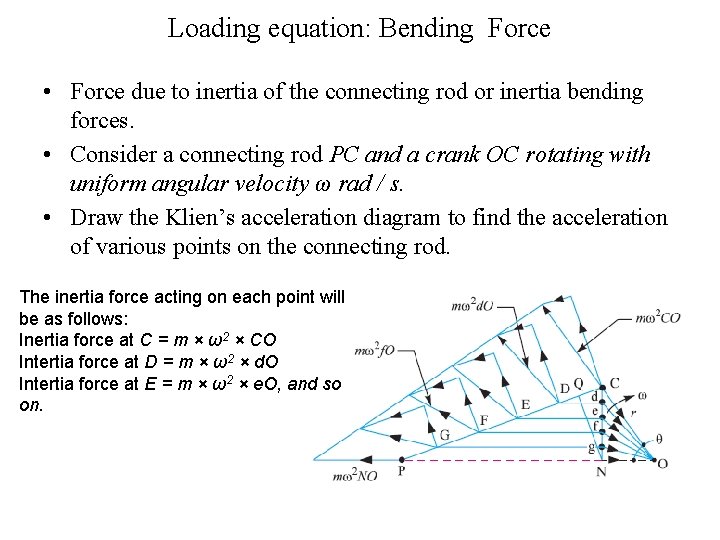
Loading equation: Bending Force • Force due to inertia of the connecting rod or inertia bending forces. • Consider a connecting rod PC and a crank OC rotating with uniform angular velocity ω rad / s. • Draw the Klien’s acceleration diagram to find the acceleration of various points on the connecting rod. The inertia force acting on each point will be as follows: Inertia force at C = m × ω2 × CO Intertia force at D = m × ω2 × d. O Intertia force at E = m × ω2 × e. O, and so on.
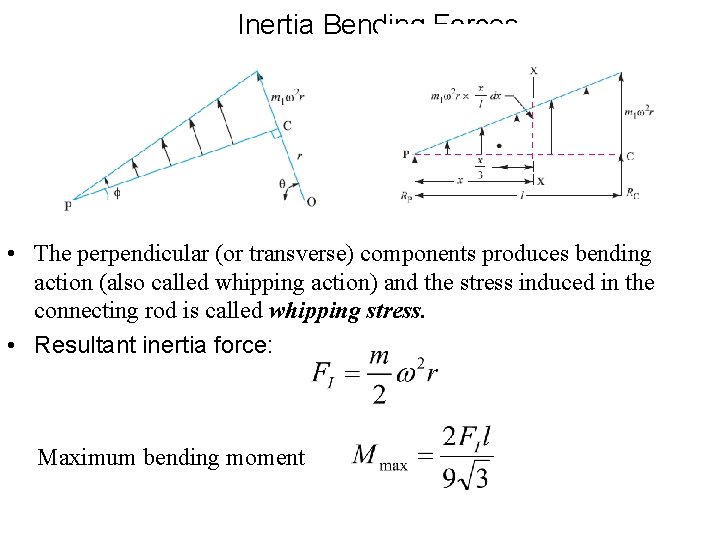
Inertia Bending Forces • The perpendicular (or transverse) components produces bending action (also called whipping action) and the stress induced in the connecting rod is called whipping stress. • Resultant inertia force: Maximum bending moment
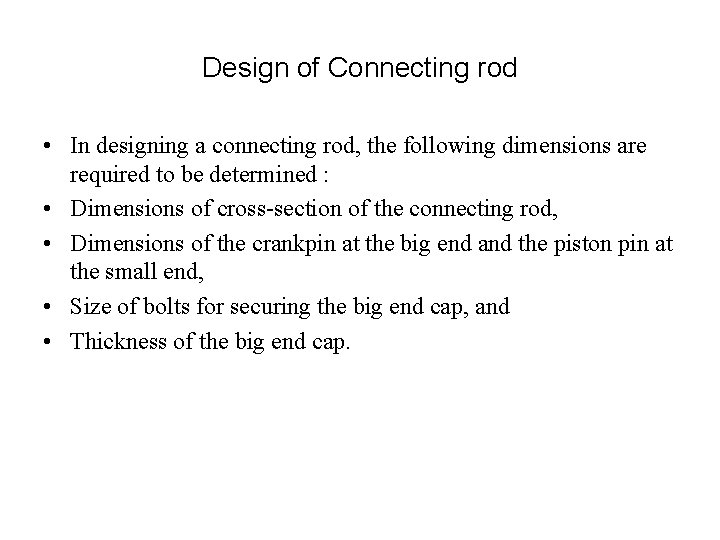
Design of Connecting rod • In designing a connecting rod, the following dimensions are required to be determined : • Dimensions of cross-section of the connecting rod, • Dimensions of the crankpin at the big end and the piston pin at the small end, • Size of bolts for securing the big end cap, and • Thickness of the big end cap.
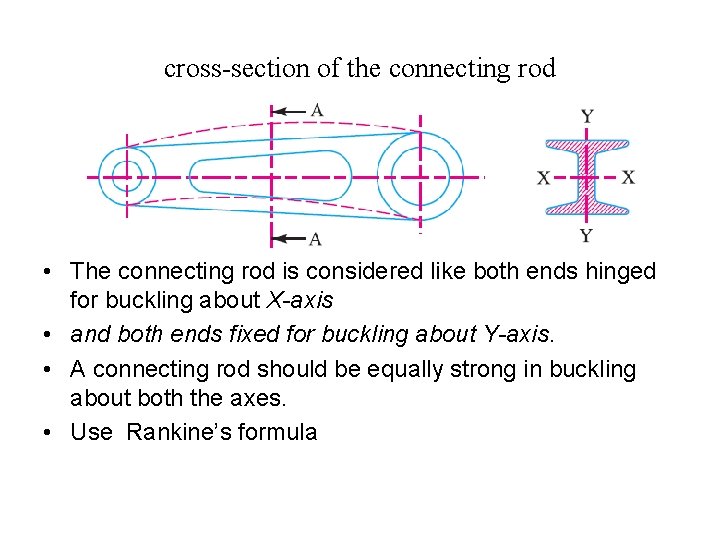
cross-section of the connecting rod • The connecting rod is considered like both ends hinged for buckling about X-axis • and both ends fixed for buckling about Y-axis. • A connecting rod should be equally strong in buckling about both the axes. • Use Rankine’s formula
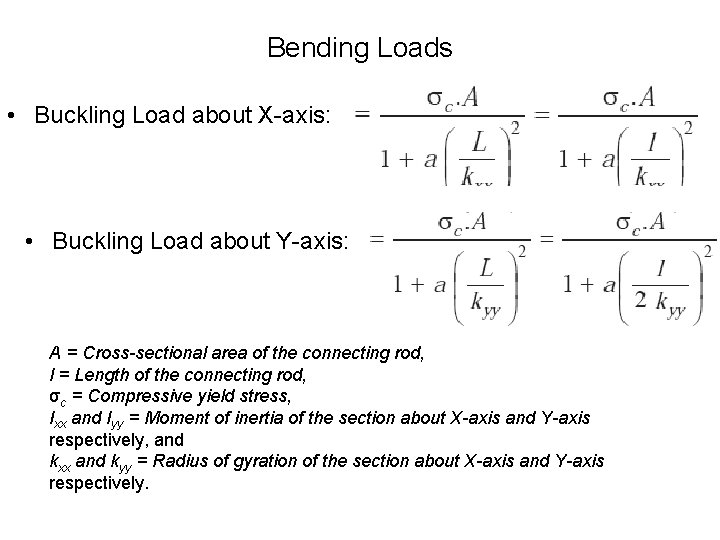
Bending Loads • Buckling Load about X-axis: • Buckling Load about Y-axis: A = Cross-sectional area of the connecting rod, l = Length of the connecting rod, σc = Compressive yield stress, Ixx and Iyy = Moment of inertia of the section about X-axis and Y-axis respectively, and kxx and kyy = Radius of gyration of the section about X-axis and Y-axis respectively.
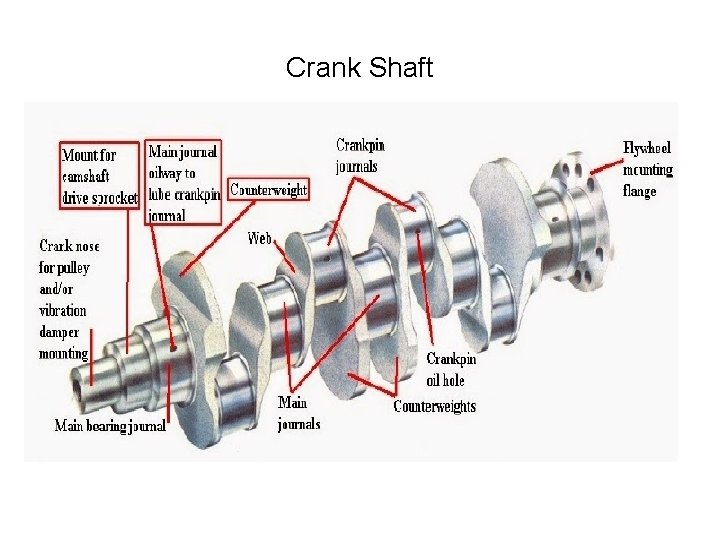
Crank Shaft
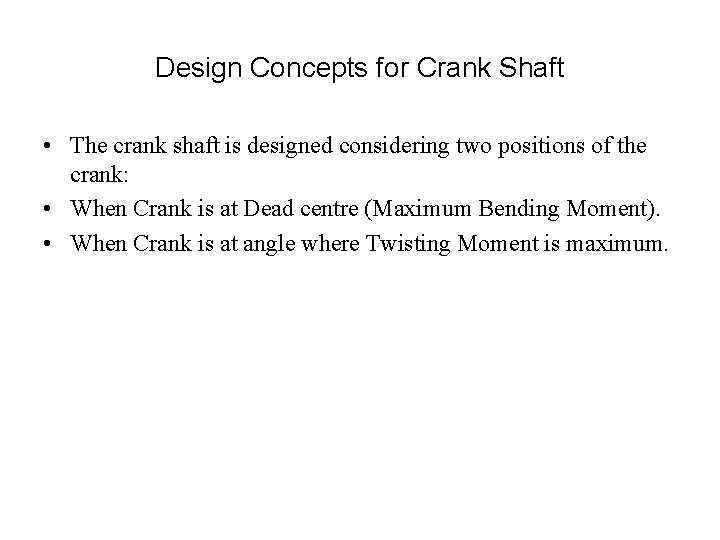
Design Concepts for Crank Shaft • The crank shaft is designed considering two positions of the crank: • When Crank is at Dead centre (Maximum Bending Moment). • When Crank is at angle where Twisting Moment is maximum.
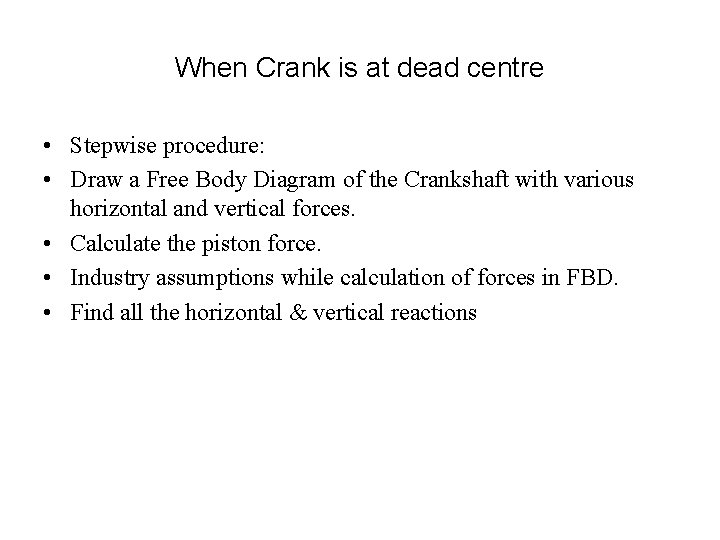
When Crank is at dead centre • Stepwise procedure: • Draw a Free Body Diagram of the Crankshaft with various horizontal and vertical forces. • Calculate the piston force. • Industry assumptions while calculation of forces in FBD. • Find all the horizontal & vertical reactions
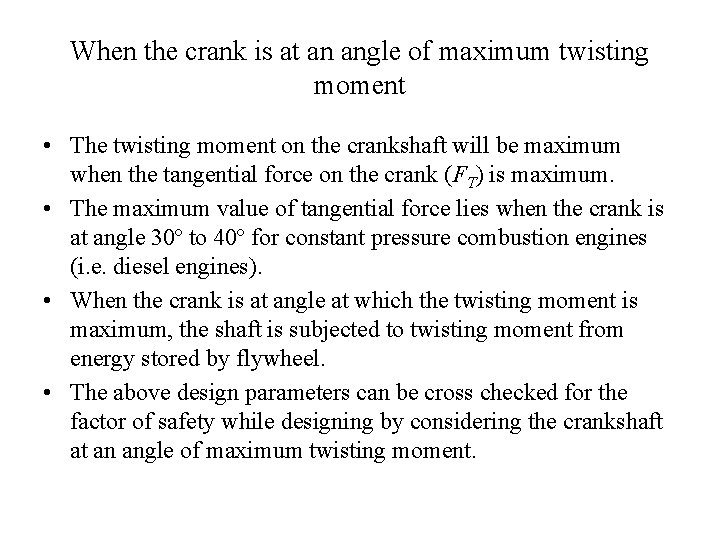
When the crank is at an angle of maximum twisting moment • The twisting moment on the crankshaft will be maximum when the tangential force on the crank (FT) is maximum. • The maximum value of tangential force lies when the crank is at angle 30º to 40º for constant pressure combustion engines (i. e. diesel engines). • When the crank is at angle at which the twisting moment is maximum, the shaft is subjected to twisting moment from energy stored by flywheel. • The above design parameters can be cross checked for the factor of safety while designing by considering the crankshaft at an angle of maximum twisting moment.
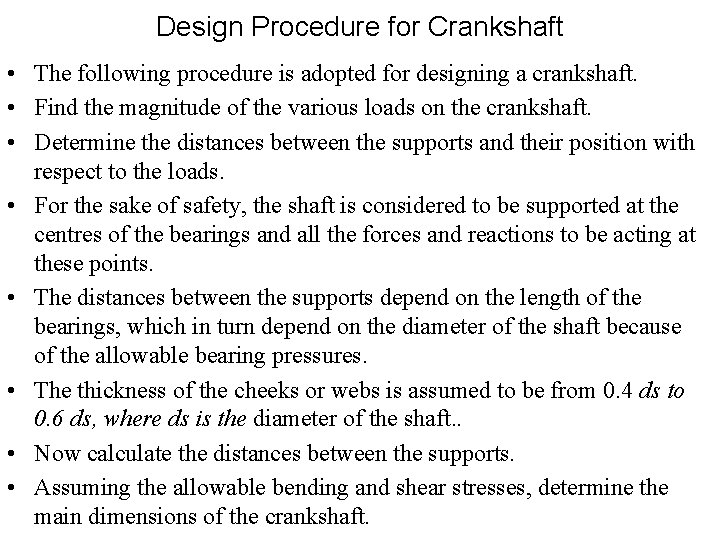
Design Procedure for Crankshaft • The following procedure is adopted for designing a crankshaft. • Find the magnitude of the various loads on the crankshaft. • Determine the distances between the supports and their position with respect to the loads. • For the sake of safety, the shaft is considered to be supported at the centres of the bearings and all the forces and reactions to be acting at these points. • The distances between the supports depend on the length of the bearings, which in turn depend on the diameter of the shaft because of the allowable bearing pressures. • The thickness of the cheeks or webs is assumed to be from 0. 4 ds to 0. 6 ds, where ds is the diameter of the shaft. . • Now calculate the distances between the supports. • Assuming the allowable bending and shear stresses, determine the main dimensions of the crankshaft.
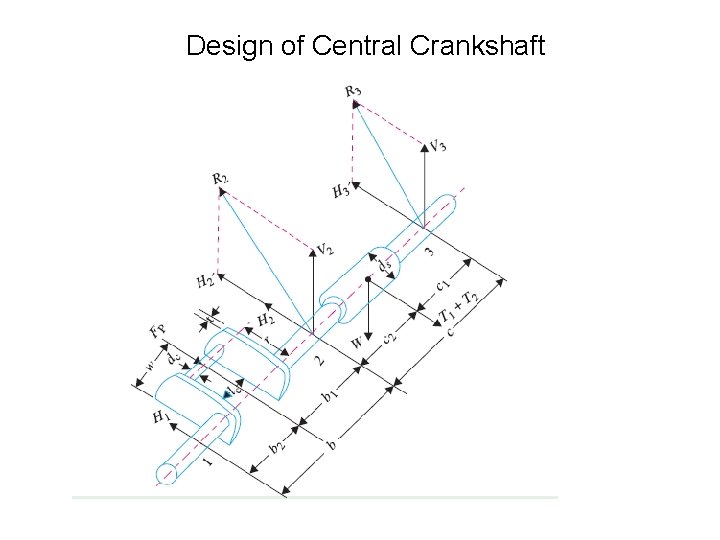
Design of Central Crankshaft
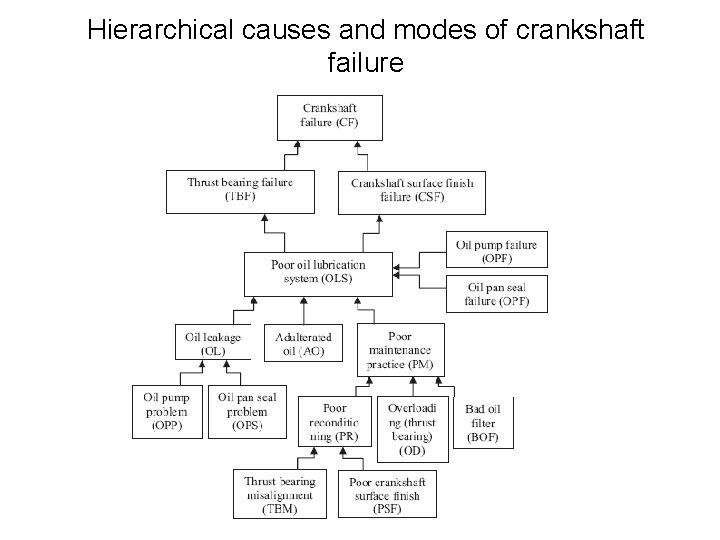
Hierarchical causes and modes of crankshaft failure
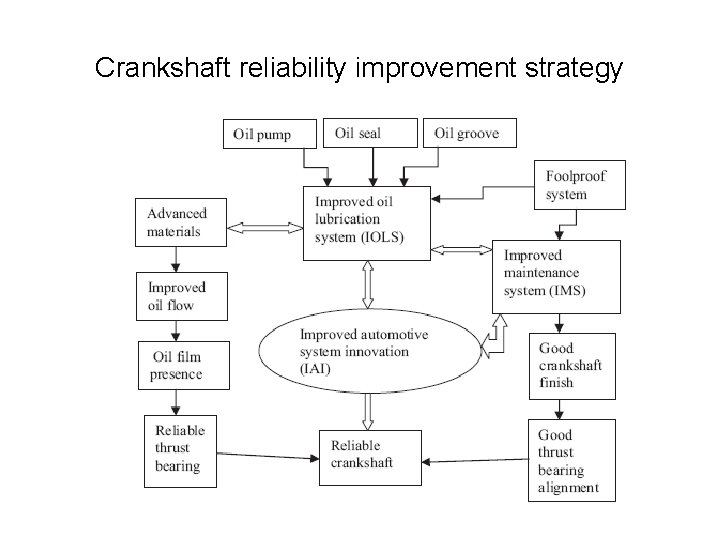
Crankshaft reliability improvement strategy
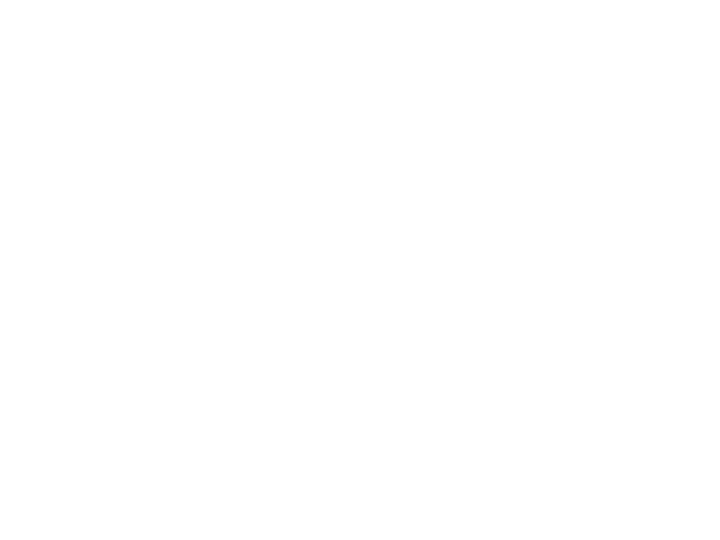
Mechanical engine parts
Internal combustion vs external combustion
Ip*3600/(mf*cv) is
Actual mechanical advantage vs ideal mechanical advantage
Engine parts identification test
Engine part quiz
Bus engine parts name
Small engine block
Small engine parts identification
Light microscope parts and functions
Mechanical wave parts
Magnifying illuminating and mechanical parts of microscope
Frc chassis
Rotating disc where the objectives are attached
Combustion analysis in engine design
Spark design mechanical
What is machine
Hydraulic flocculator
Mechanical engineering design book
Mechanical considerations of transmission line
Machine elements in mechanical design 5th edition
Proportion definition in floral design
Mechanical housing design
Eric montesinos
Cold box design
Mechanical process equipment
Machine elements in mechanical design
Mechanical
Mechanical design
Mechanical design
Mechanical engineering design
Mechanical engineering design
Part of business letter
Fraction unequal parts
Six chief parts of the small catechism
Rhythm by gradation in interior design
Name the two parts of a table design screen
Worlds largest engine
How much work is done in holding a 15 n sack of potatoes
Whats a search engine
What is jsp
How much is asi membership
Microsoft rules engine
Introduction to sketch
Performance curve of ic engine
Light tanker for supplying water or fuel
How does a jet engine work animation
Tugas dari web engine adalah
General engine diagnosis
Volumetric efficiency formula
Trent 700 engine diagram
Rotary engine disadvantages
Goto search engine
The anatomy of a large scale hypertextual web search engine
The anatomy of a large-scale hypertextual web search engine
Test information distribution engine (tide)
Sustainable and green engines
Supercharging of ic engine
Coil steam engine
Difference between web browser and search engine
Social value engine