Chapter 18 Electrochemistry OxidationReduction REDOX Reactions in which
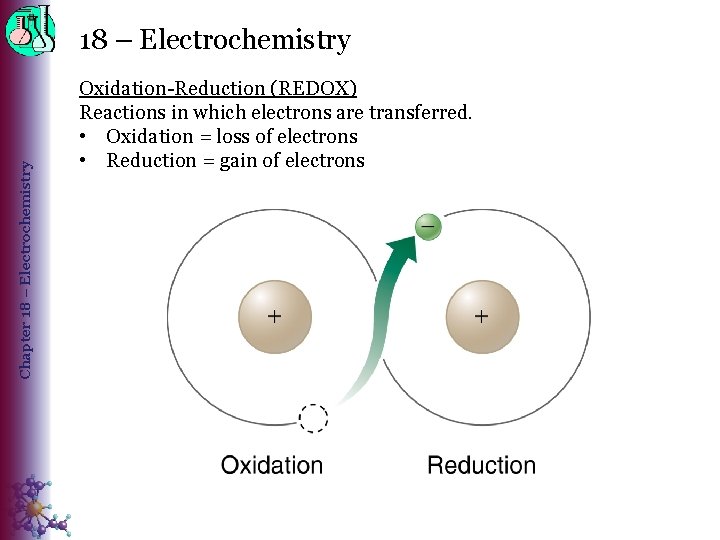
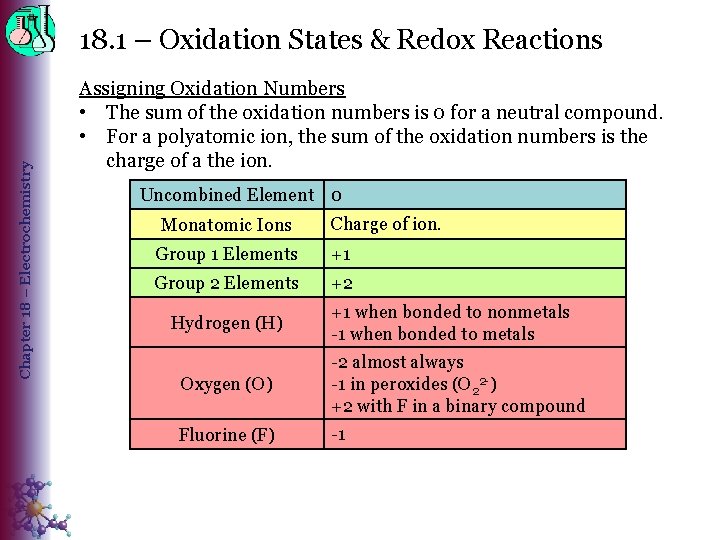
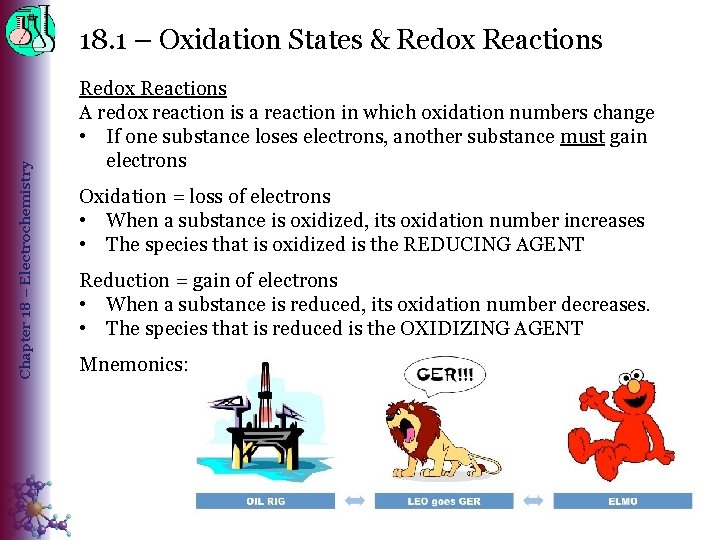
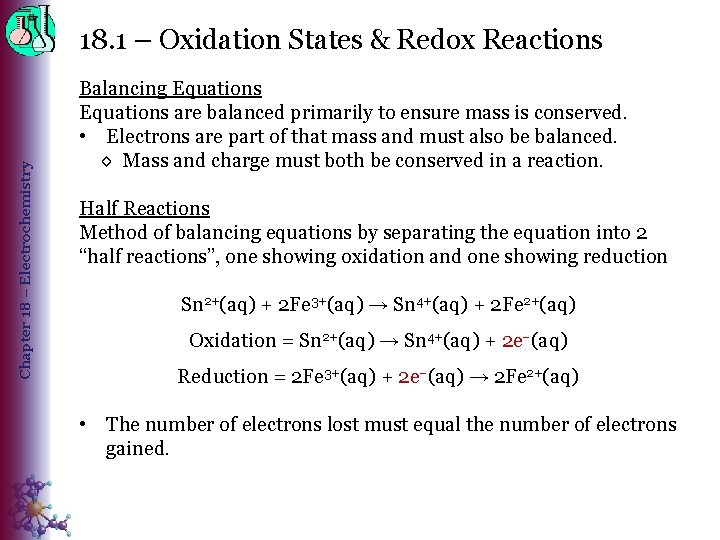
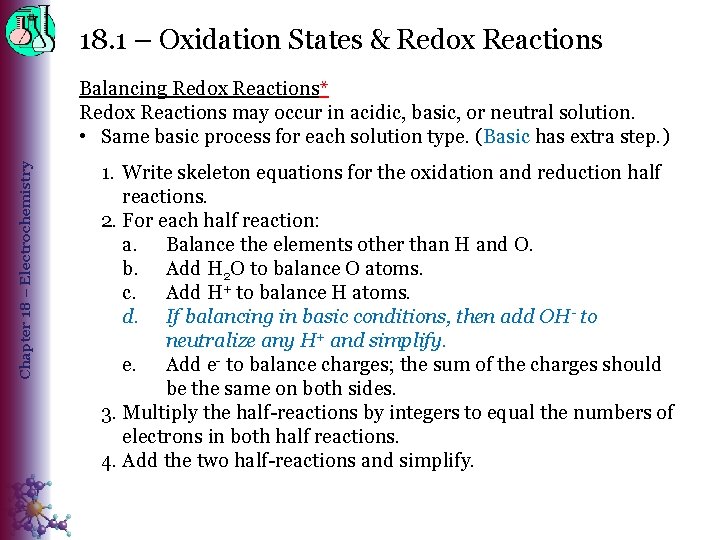
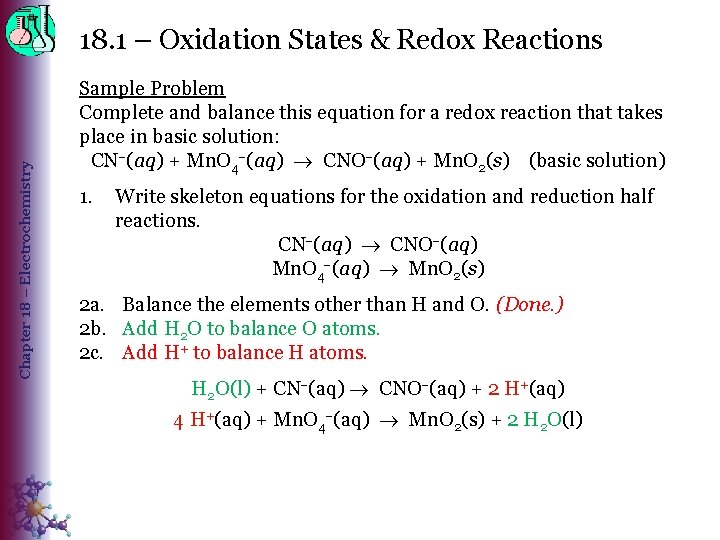
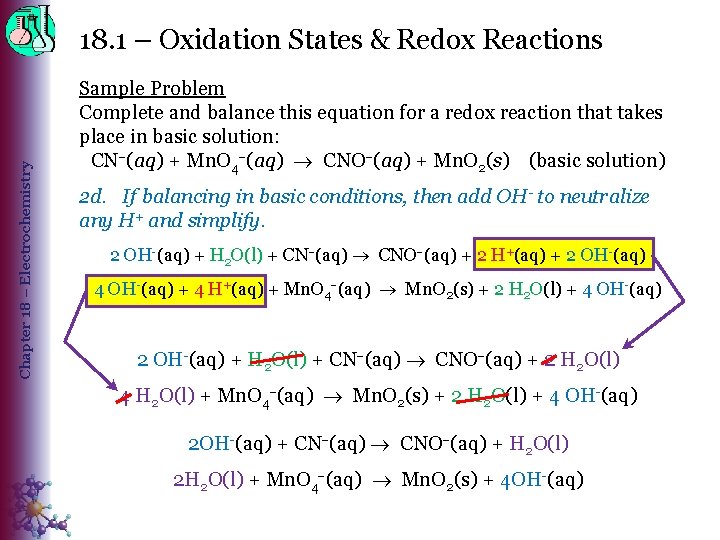
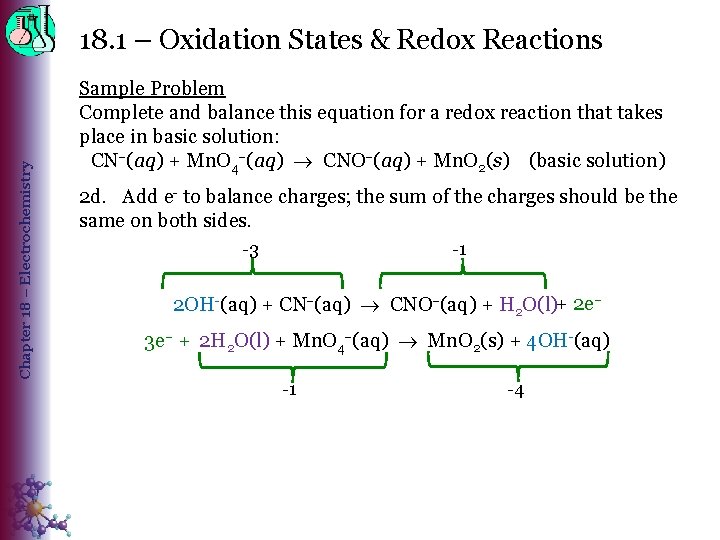
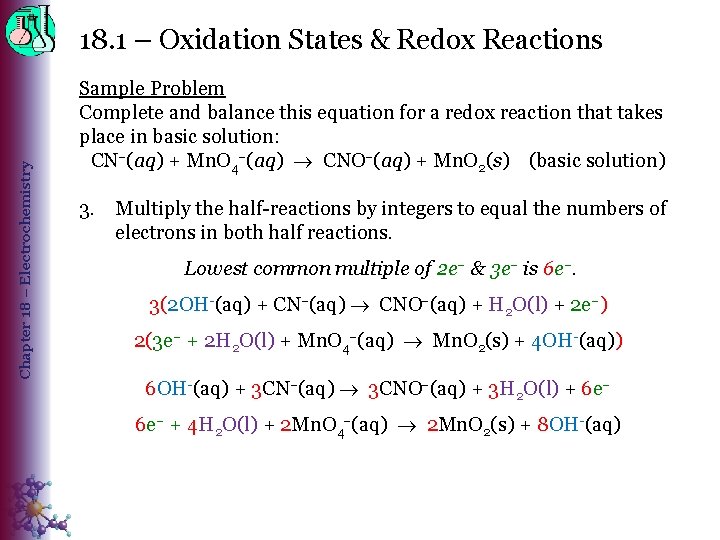
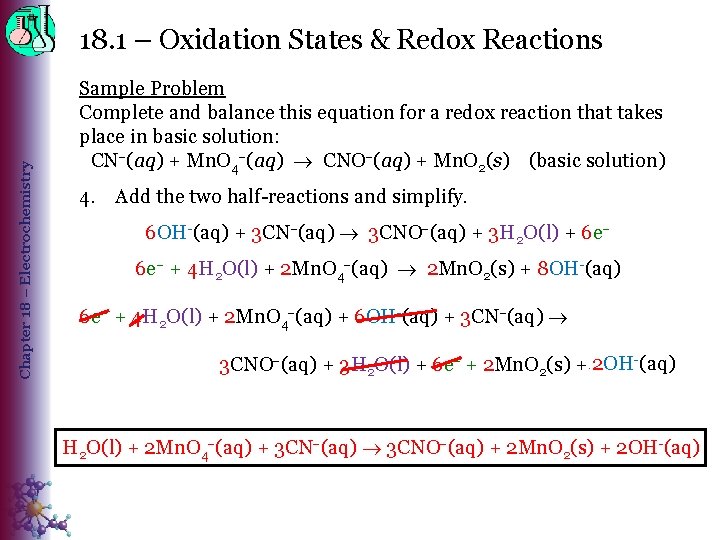
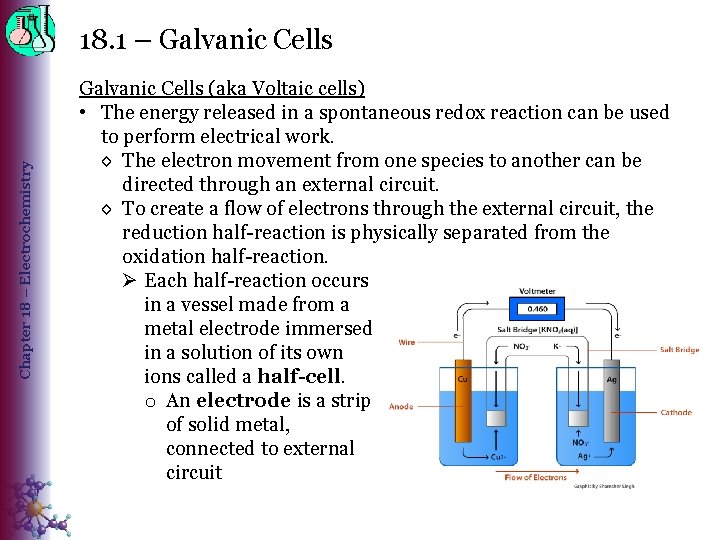
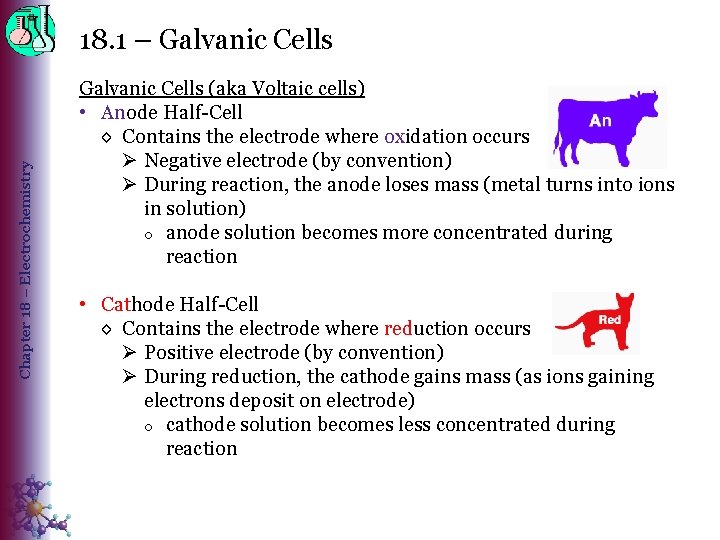
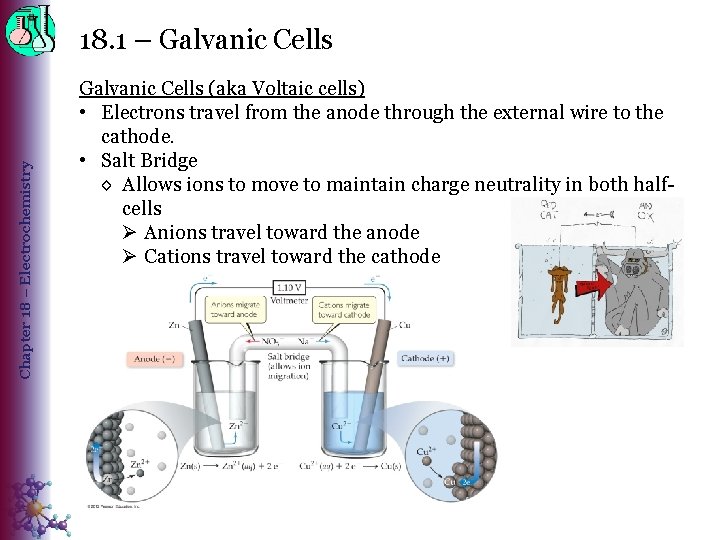
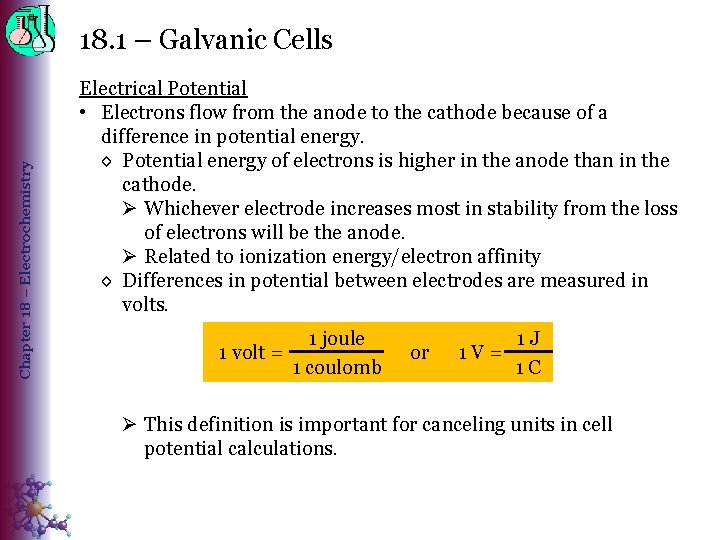
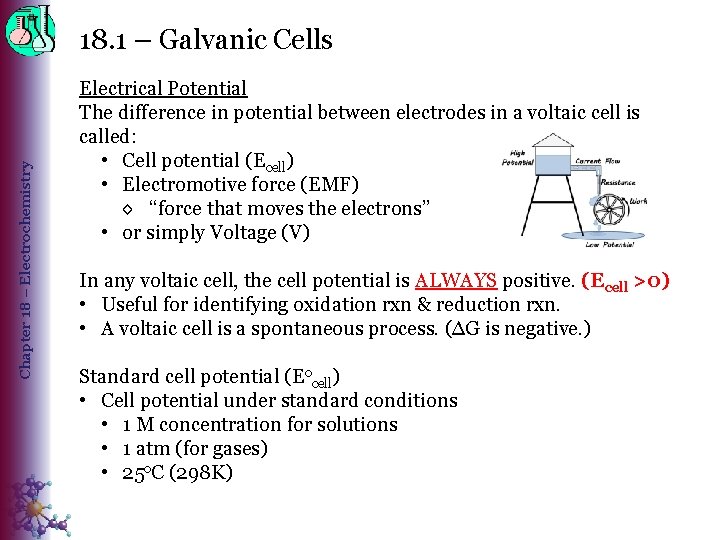
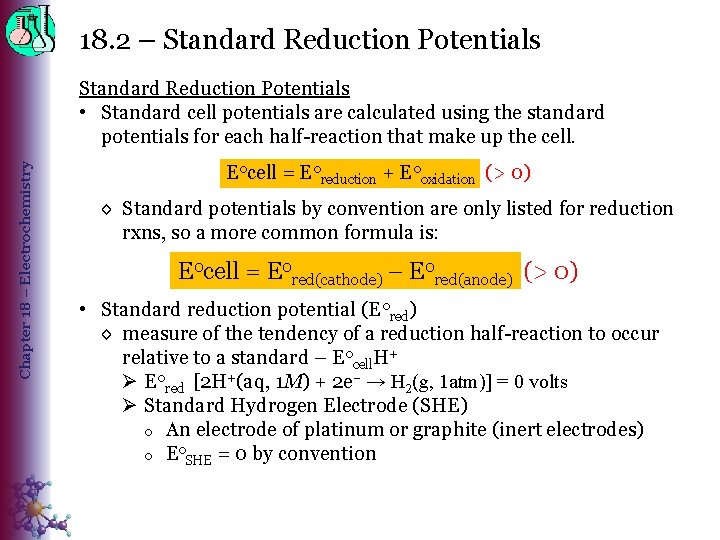
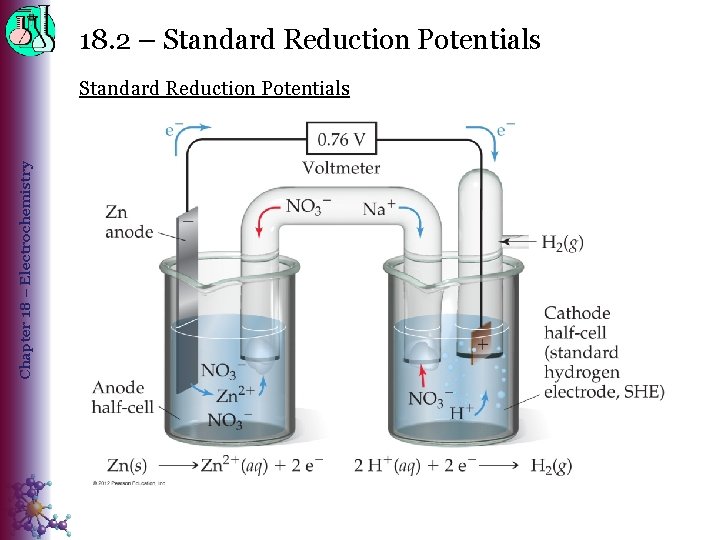
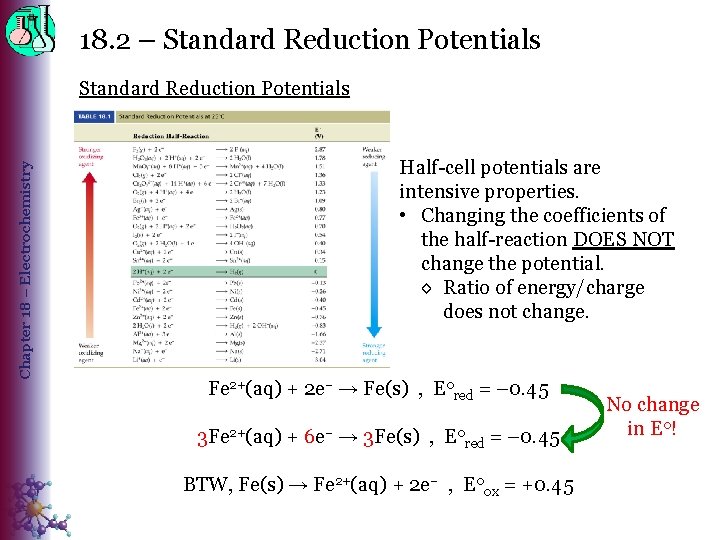
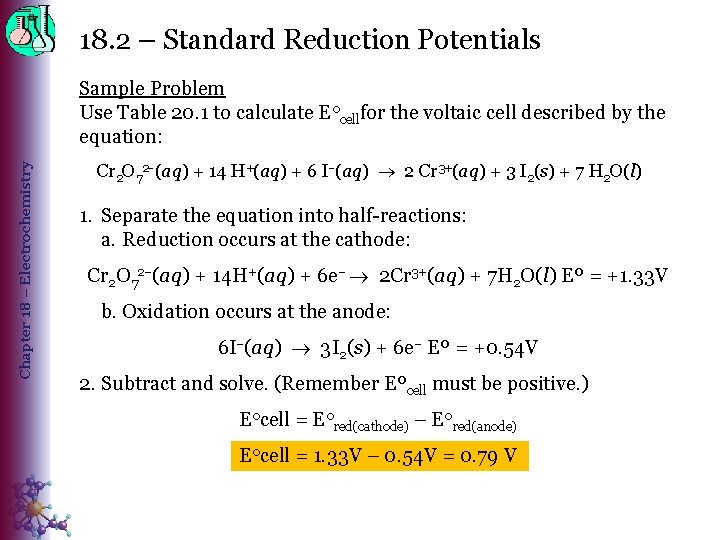
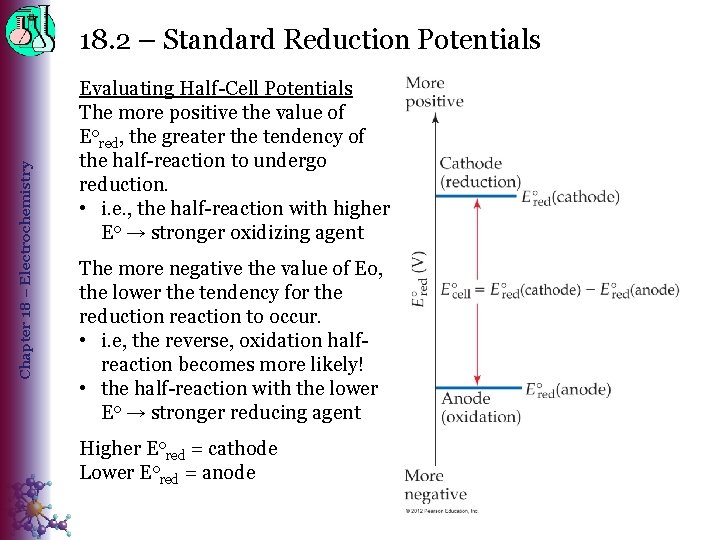
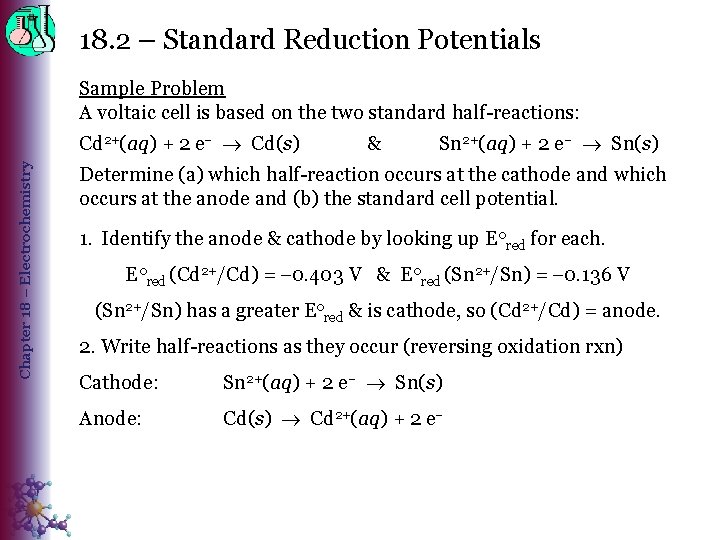
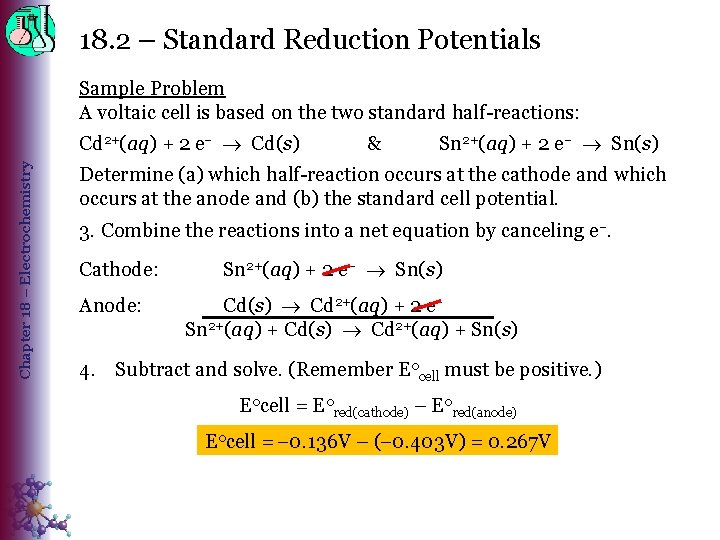
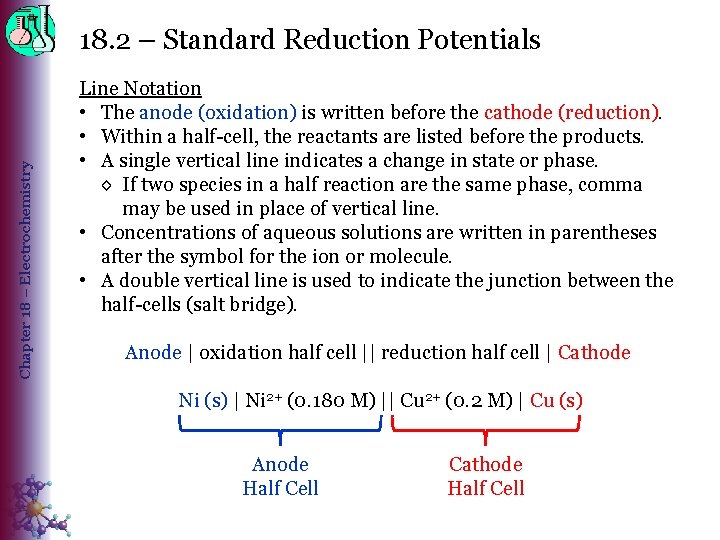
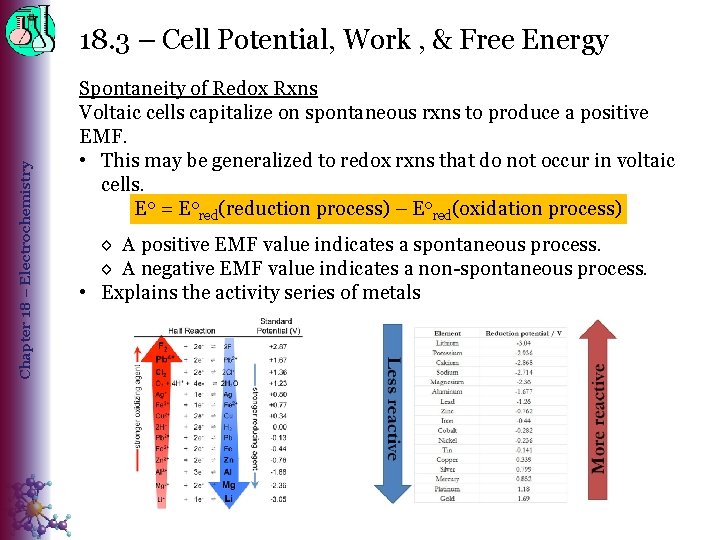
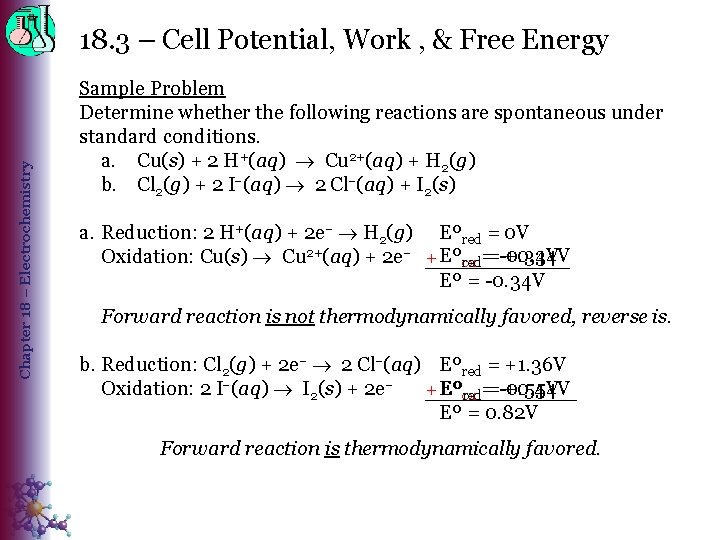
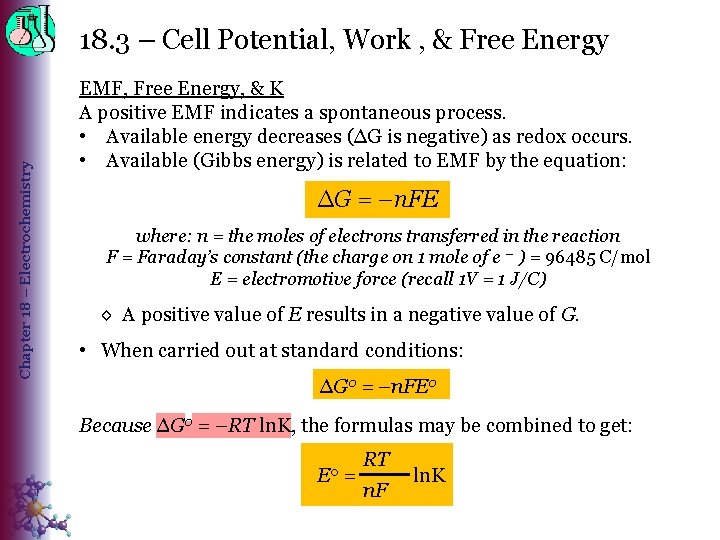
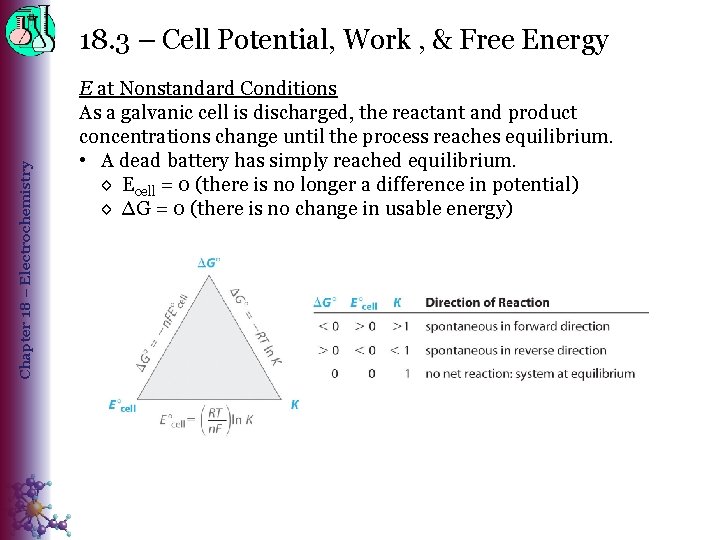
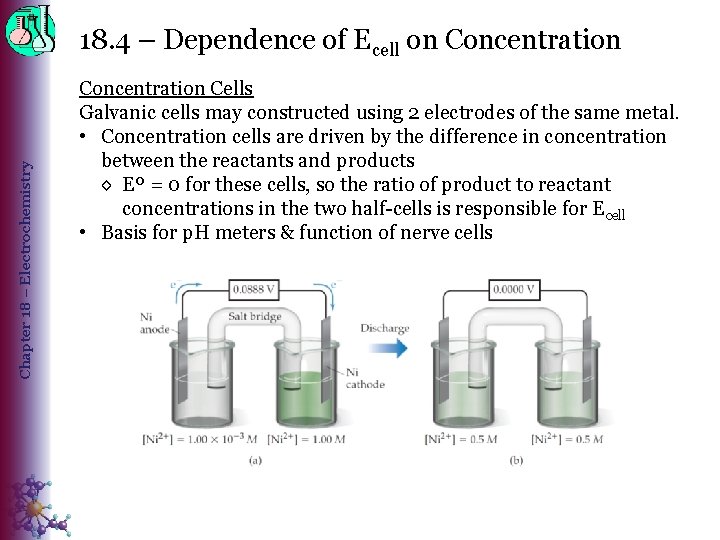
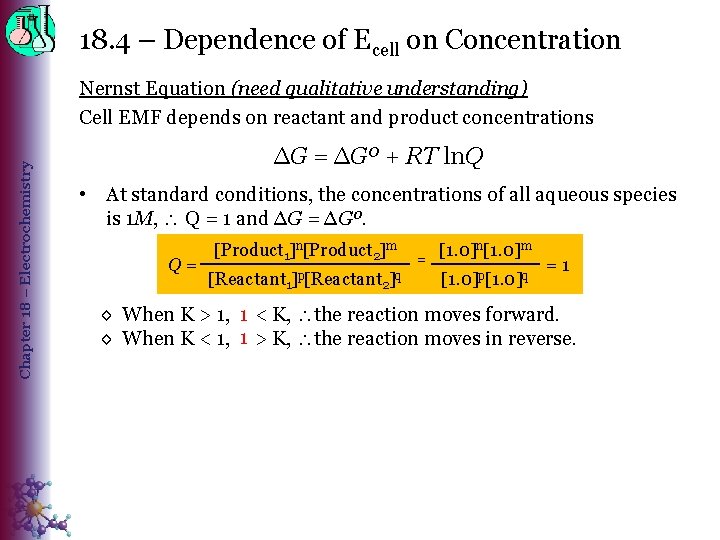
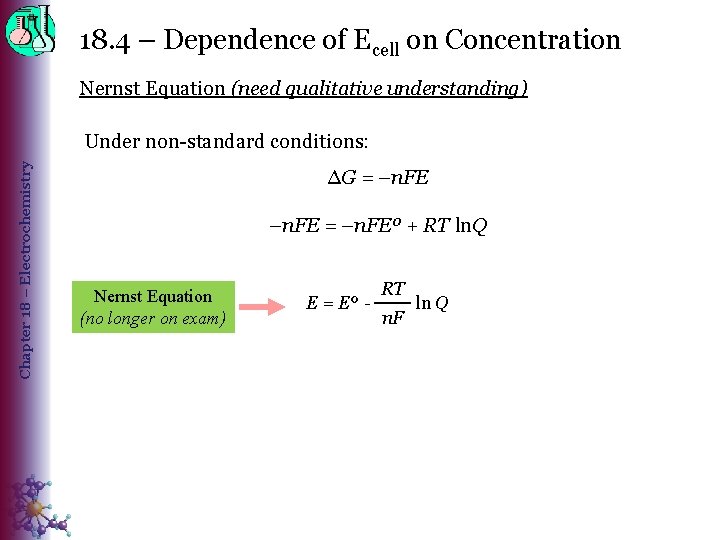
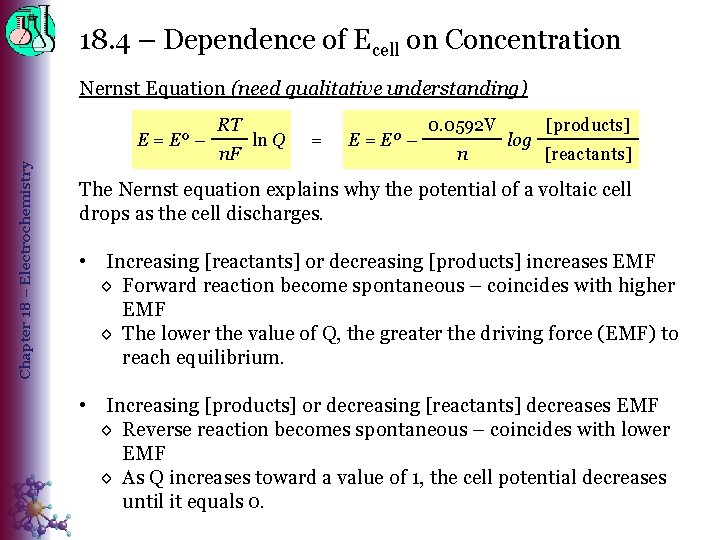
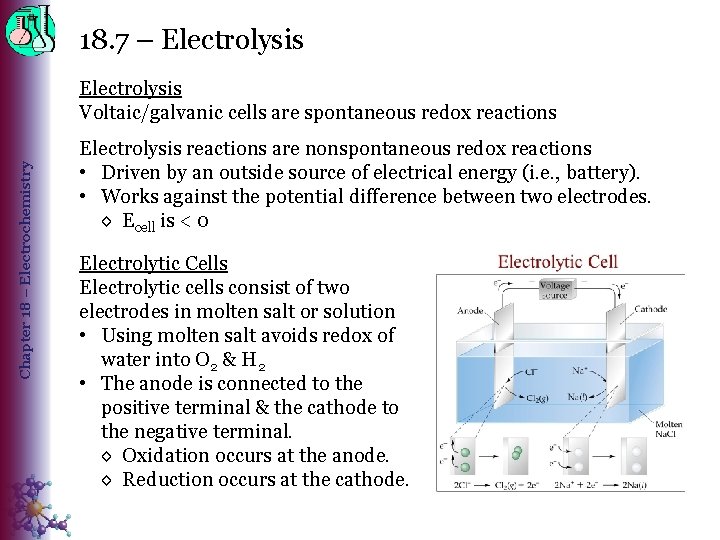
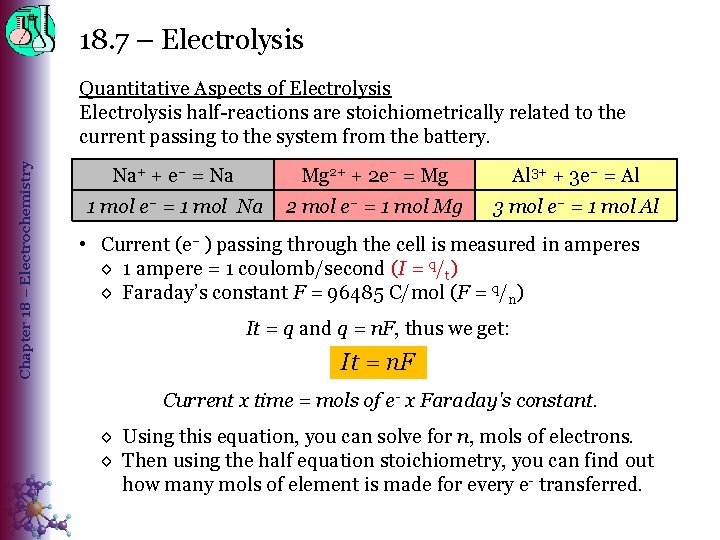
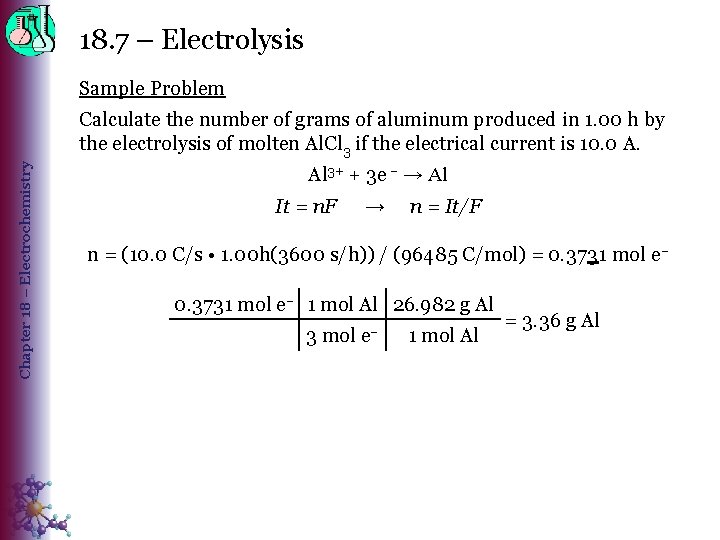
- Slides: 34
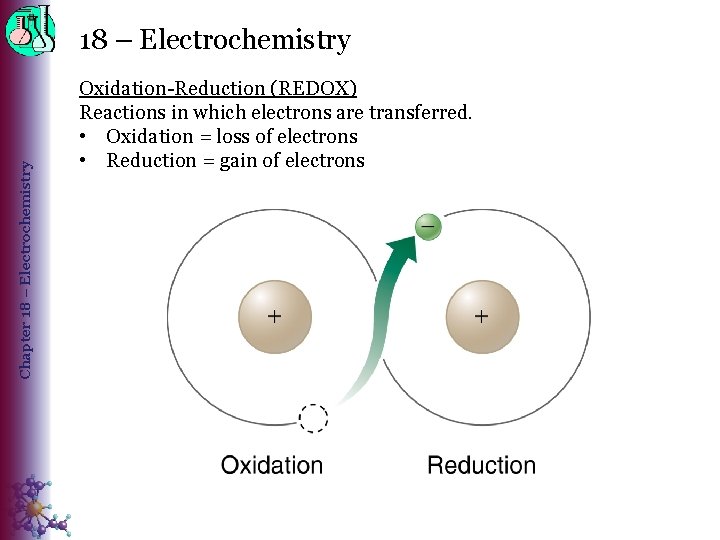
Chapter 18 – Electrochemistry Oxidation-Reduction (REDOX) Reactions in which electrons are transferred. • Oxidation = loss of electrons • Reduction = gain of electrons
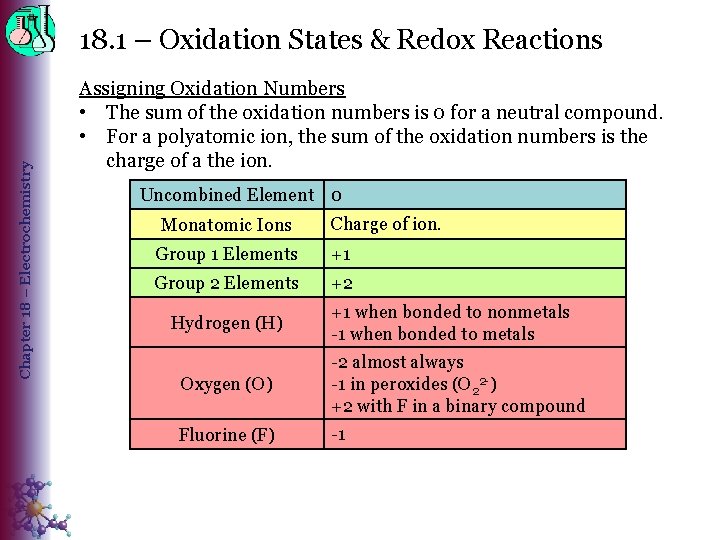
Chapter 18 – Electrochemistry 18. 1 – Oxidation States & Redox Reactions Assigning Oxidation Numbers • The sum of the oxidation numbers is 0 for a neutral compound. • For a polyatomic ion, the sum of the oxidation numbers is the charge of a the ion. Uncombined Element 0 Monatomic Ions Charge of ion. Group 1 Elements +1 Group 2 Elements +2 Hydrogen (H) +1 when bonded to nonmetals -1 when bonded to metals Oxygen (O) -2 almost always -1 in peroxides (O 22 -) +2 with F in a binary compound Fluorine (F) -1
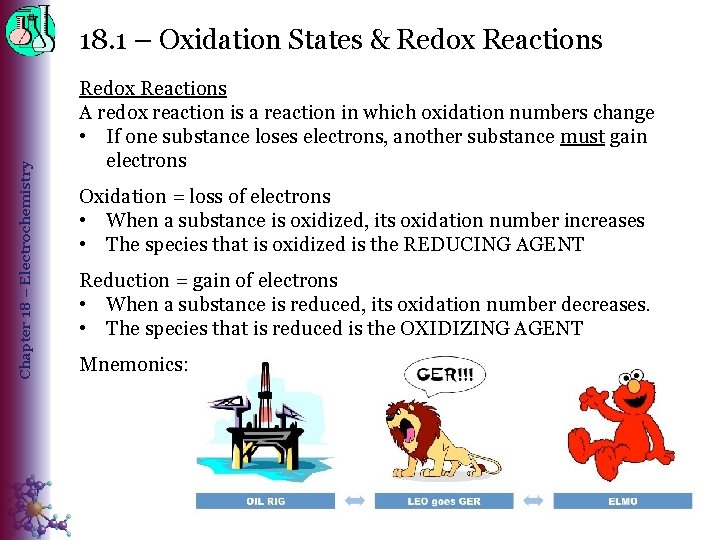
Chapter 18 – Electrochemistry 18. 1 – Oxidation States & Redox Reactions A redox reaction is a reaction in which oxidation numbers change • If one substance loses electrons, another substance must gain electrons Oxidation = loss of electrons • When a substance is oxidized, its oxidation number increases • The species that is oxidized is the REDUCING AGENT Reduction = gain of electrons • When a substance is reduced, its oxidation number decreases. • The species that is reduced is the OXIDIZING AGENT Mnemonics:
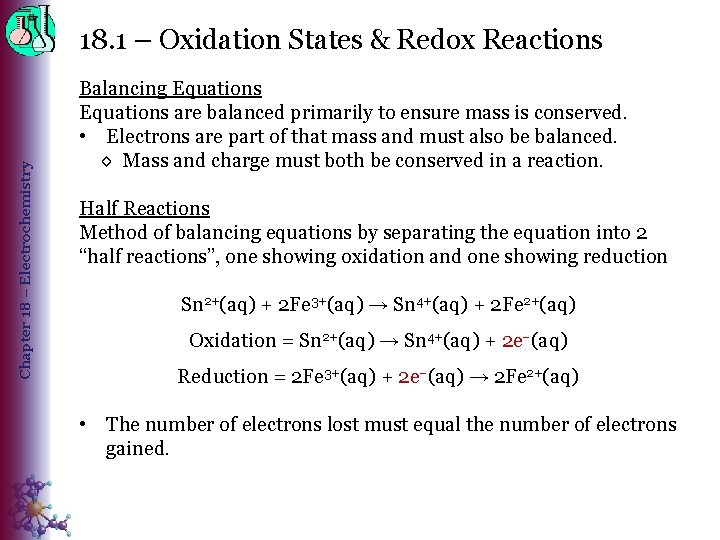
Chapter 18 – Electrochemistry 18. 1 – Oxidation States & Redox Reactions Balancing Equations are balanced primarily to ensure mass is conserved. • Electrons are part of that mass and must also be balanced. ◊ Mass and charge must both be conserved in a reaction. Half Reactions Method of balancing equations by separating the equation into 2 “half reactions”, one showing oxidation and one showing reduction Sn 2+(aq) + 2 Fe 3+(aq) → Sn 4+(aq) + 2 Fe 2+(aq) Oxidation = Sn 2+(aq) → Sn 4+(aq) + 2 e–(aq) Reduction = 2 Fe 3+(aq) + 2 e–(aq) → 2 Fe 2+(aq) • The number of electrons lost must equal the number of electrons gained.
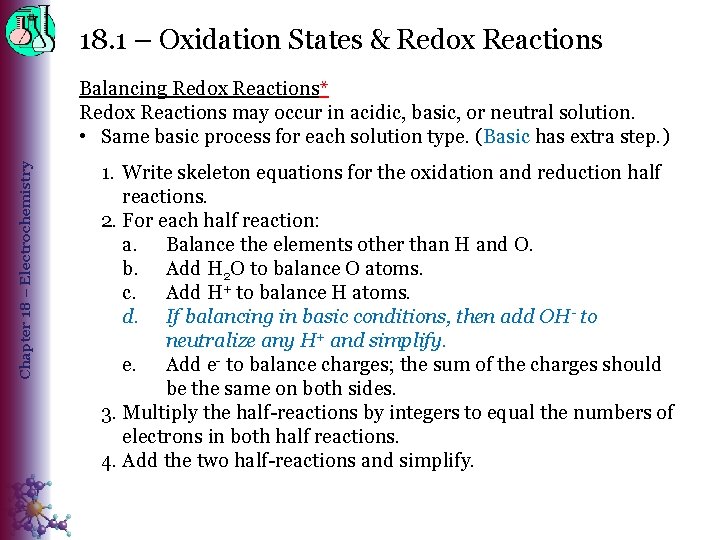
18. 1 – Oxidation States & Redox Reactions Chapter 18 – Electrochemistry Balancing Redox Reactions* Redox Reactions may occur in acidic, basic, or neutral solution. • Same basic process for each solution type. (Basic has extra step. ) 1. Write skeleton equations for the oxidation and reduction half reactions. 2. For each half reaction: a. Balance the elements other than H and O. b. Add H 2 O to balance O atoms. c. Add H+ to balance H atoms. d. If balancing in basic conditions, then add OH- to neutralize any H+ and simplify. e. Add e- to balance charges; the sum of the charges should be the same on both sides. 3. Multiply the half-reactions by integers to equal the numbers of electrons in both half reactions. 4. Add the two half-reactions and simplify.
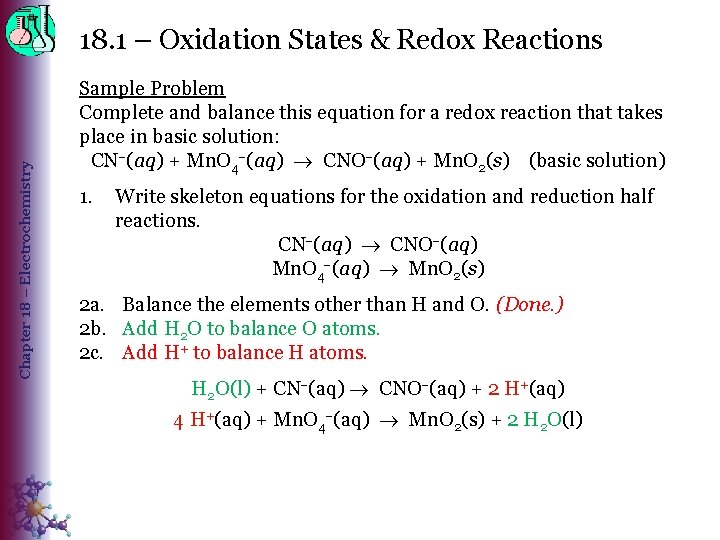
Chapter 18 – Electrochemistry 18. 1 – Oxidation States & Redox Reactions Sample Problem Complete and balance this equation for a redox reaction that takes place in basic solution: CN (aq) + Mn. O 4 (aq) CNO (aq) + Mn. O 2(s) (basic solution) 1. Write skeleton equations for the oxidation and reduction half reactions. CN (aq) CNO (aq) Mn. O 4 (aq) Mn. O 2(s) 2 a. Balance the elements other than H and O. (Done. ) 2 b. Add H 2 O to balance O atoms. 2 c. Add H+ to balance H atoms. H 2 O(l) + CN (aq) CNO (aq) + 2 H+(aq) 4 H+(aq) + Mn. O 4 (aq) Mn. O 2(s) + 2 H 2 O(l)
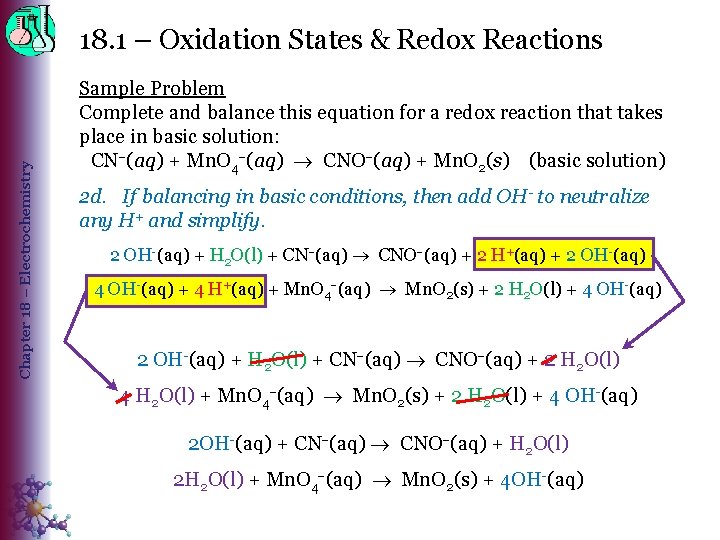
Chapter 18 – Electrochemistry 18. 1 – Oxidation States & Redox Reactions Sample Problem Complete and balance this equation for a redox reaction that takes place in basic solution: CN (aq) + Mn. O 4 (aq) CNO (aq) + Mn. O 2(s) (basic solution) 2 d. If balancing in basic conditions, then add OH- to neutralize any H+ and simplify. 2 OH-(aq) + H 2 O(l) + CN (aq) CNO (aq) + 2 H+(aq) + 2 OH-(aq) 4 OH-(aq) + 4 H+(aq) + Mn. O 4 (aq) Mn. O 2(s) + 2 H 2 O(l) + 4 OH-(aq) 2 OH-(aq) + H 2 O(l) + CN (aq) CNO (aq) + 2 H 2 O(l) 4 H 2 O(l) + Mn. O 4 (aq) Mn. O 2(s) + 2 H 2 O(l) + 4 OH-(aq) 2 OH-(aq) + CN (aq) CNO (aq) + H 2 O(l) 2 H 2 O(l) + Mn. O 4 (aq) Mn. O 2(s) + 4 OH-(aq)
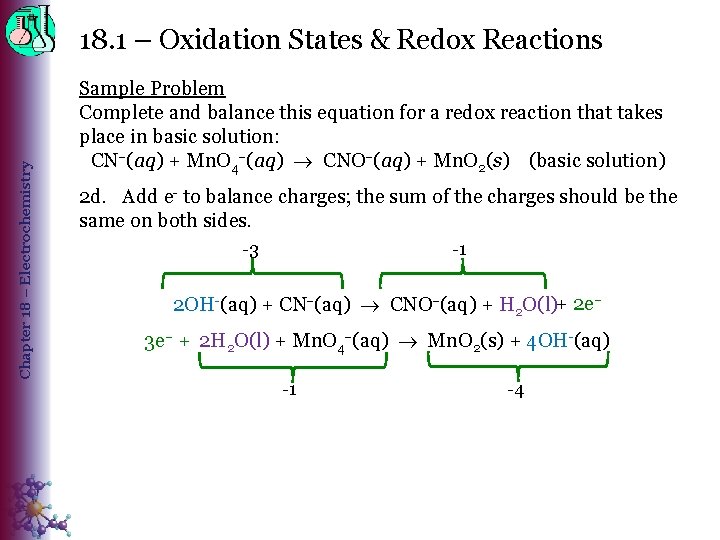
Chapter 18 – Electrochemistry 18. 1 – Oxidation States & Redox Reactions Sample Problem Complete and balance this equation for a redox reaction that takes place in basic solution: CN (aq) + Mn. O 4 (aq) CNO (aq) + Mn. O 2(s) (basic solution) 2 d. Add e- to balance charges; the sum of the charges should be the same on both sides. -3 -1 2 OH-(aq) + CN (aq) CNO (aq) + H 2 O(l)+ 2 e– 3 e– + 2 H 2 O(l) + Mn. O 4 (aq) Mn. O 2(s) + 4 OH-(aq) -1 -4
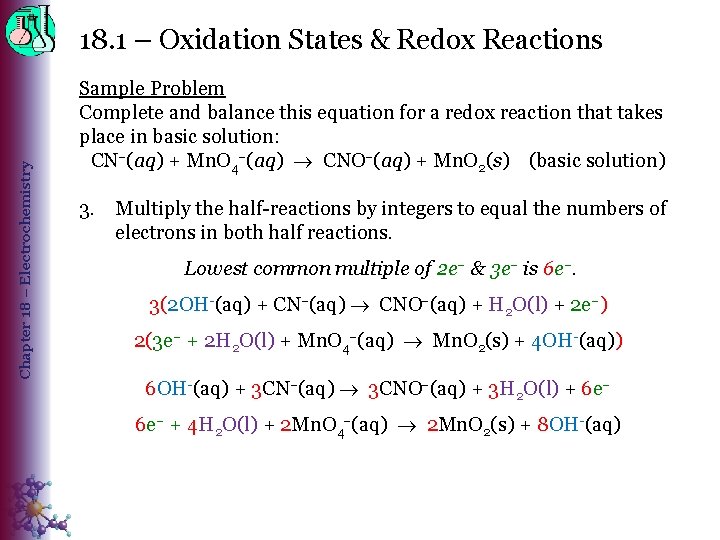
Chapter 18 – Electrochemistry 18. 1 – Oxidation States & Redox Reactions Sample Problem Complete and balance this equation for a redox reaction that takes place in basic solution: CN (aq) + Mn. O 4 (aq) CNO (aq) + Mn. O 2(s) (basic solution) 3. Multiply the half-reactions by integers to equal the numbers of electrons in both half reactions. Lowest common multiple of 2 e– & 3 e– is 6 e–. 3(2 OH-(aq) + CN (aq) CNO (aq) + H 2 O(l) + 2 e–) 2(3 e– + 2 H 2 O(l) + Mn. O 4 (aq) Mn. O 2(s) + 4 OH-(aq)) 6 OH-(aq) + 3 CN (aq) 3 CNO (aq) + 3 H 2 O(l) + 6 e– + 4 H 2 O(l) + 2 Mn. O 4 (aq) 2 Mn. O 2(s) + 8 OH-(aq)
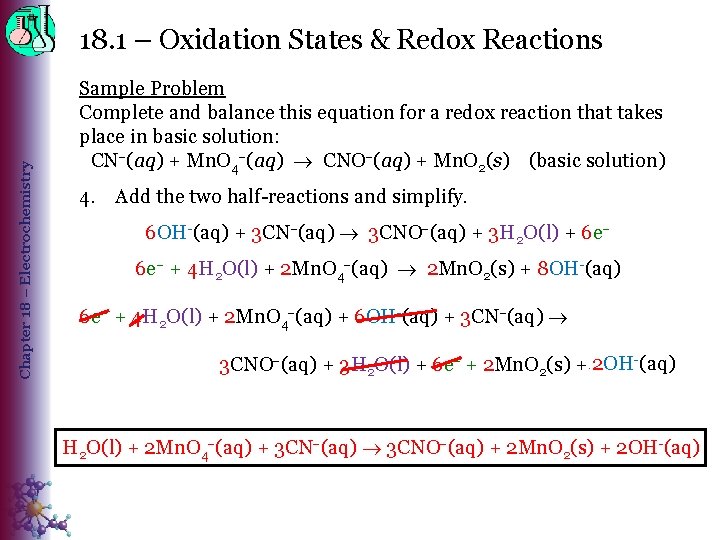
Chapter 18 – Electrochemistry 18. 1 – Oxidation States & Redox Reactions Sample Problem Complete and balance this equation for a redox reaction that takes place in basic solution: CN (aq) + Mn. O 4 (aq) CNO (aq) + Mn. O 2(s) (basic solution) 4. Add the two half-reactions and simplify. 6 OH-(aq) + 3 CN (aq) 3 CNO (aq) + 3 H 2 O(l) + 6 e– + 4 H 2 O(l) + 2 Mn. O 4 (aq) 2 Mn. O 2(s) + 8 OH-(aq) 6 e– + 4 H 2 O(l) + 2 Mn. O 4 (aq) + 6 OH-(aq) + 3 CN (aq) 3 CNO (aq) + 3 H 2 O(l) + 6 e– + 2 Mn. O 2(s) + 2 OH 8 OH--(aq) H 2 O(l) + 2 Mn. O 4 (aq) + 3 CN (aq) 3 CNO (aq) + 2 Mn. O 2(s) + 2 OH-(aq)
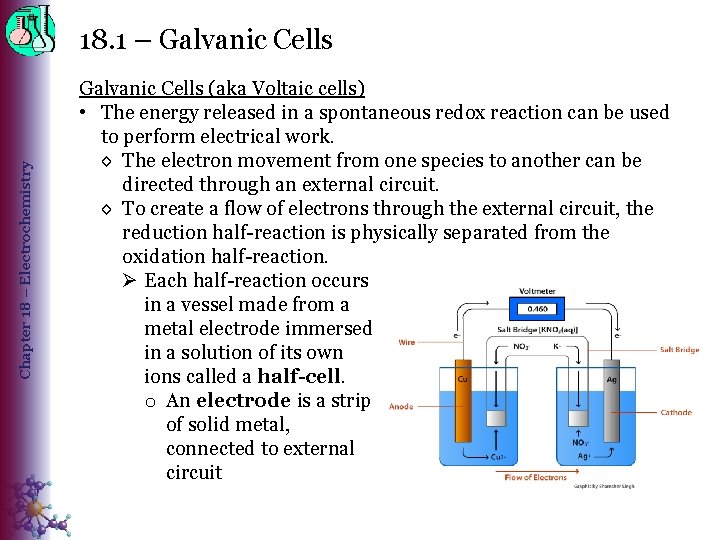
Chapter 18 – Electrochemistry 18. 1 – Galvanic Cells (aka Voltaic cells) • The energy released in a spontaneous redox reaction can be used to perform electrical work. ◊ The electron movement from one species to another can be directed through an external circuit. ◊ To create a flow of electrons through the external circuit, the reduction half-reaction is physically separated from the oxidation half-reaction. Ø Each half-reaction occurs in a vessel made from a metal electrode immersed in a solution of its own ions called a half-cell. o An electrode is a strip of solid metal, connected to external circuit
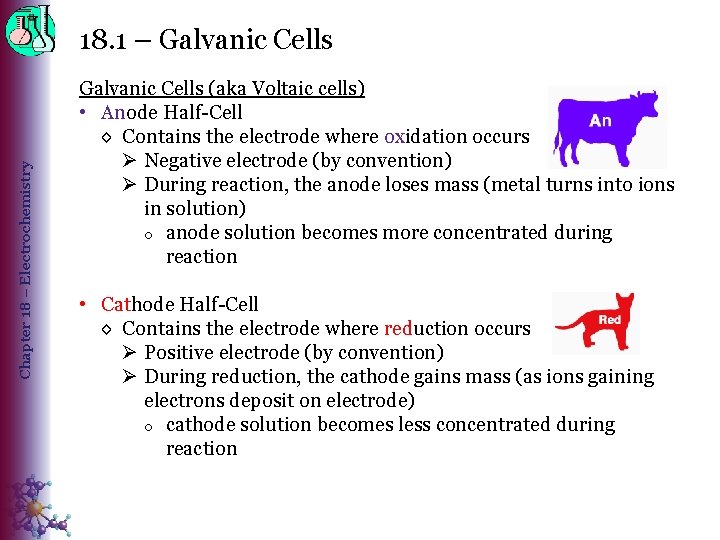
Chapter 18 – Electrochemistry 18. 1 – Galvanic Cells (aka Voltaic cells) • Anode Half-Cell ◊ Contains the electrode where oxidation occurs Ø Negative electrode (by convention) Ø During reaction, the anode loses mass (metal turns into ions in solution) o anode solution becomes more concentrated during reaction • Cathode Half-Cell ◊ Contains the electrode where reduction occurs Ø Positive electrode (by convention) Ø During reduction, the cathode gains mass (as ions gaining electrons deposit on electrode) o cathode solution becomes less concentrated during reaction
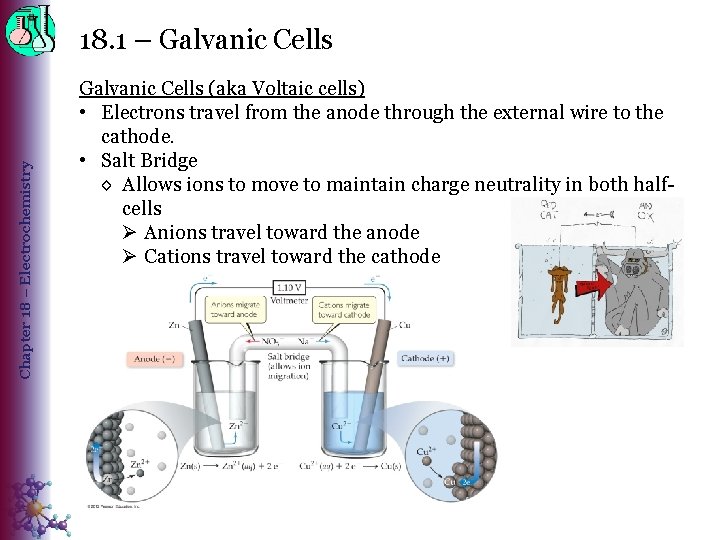
Chapter 18 – Electrochemistry 18. 1 – Galvanic Cells (aka Voltaic cells) • Electrons travel from the anode through the external wire to the cathode. • Salt Bridge ◊ Allows ions to move to maintain charge neutrality in both halfcells Ø Anions travel toward the anode Ø Cations travel toward the cathode
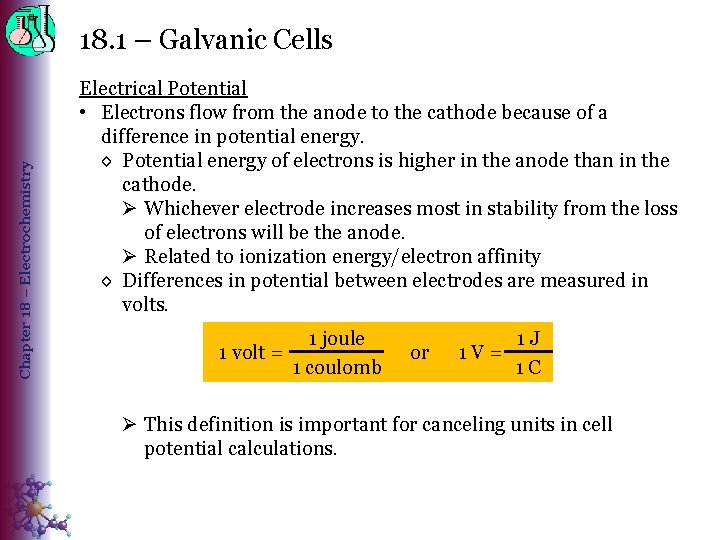
Chapter 18 – Electrochemistry 18. 1 – Galvanic Cells Electrical Potential • Electrons flow from the anode to the cathode because of a difference in potential energy. ◊ Potential energy of electrons is higher in the anode than in the cathode. Ø Whichever electrode increases most in stability from the loss of electrons will be the anode. Ø Related to ionization energy/electron affinity ◊ Differences in potential between electrodes are measured in volts. 1 volt = 1 joule 1 coulomb or 1 V= 1 J 1 C Ø This definition is important for canceling units in cell potential calculations.
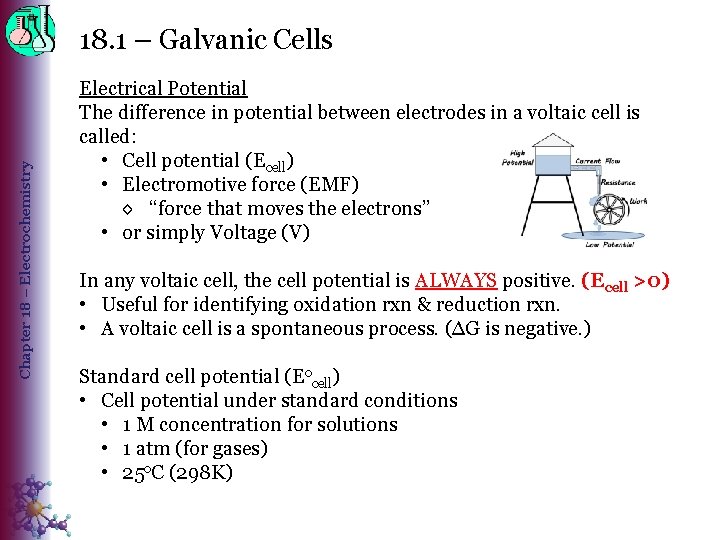
Chapter 18 – Electrochemistry 18. 1 – Galvanic Cells Electrical Potential The difference in potential between electrodes in a voltaic cell is called: • Cell potential (Ecell) • Electromotive force (EMF) ◊ “force that moves the electrons” • or simply Voltage (V) In any voltaic cell, the cell potential is ALWAYS positive. (Ecell >0) • Useful for identifying oxidation rxn & reduction rxn. • A voltaic cell is a spontaneous process. (∆G is negative. ) Standard cell potential (Eocell) • Cell potential under standard conditions • 1 M concentration for solutions • 1 atm (for gases) • 25 o. C (298 K)
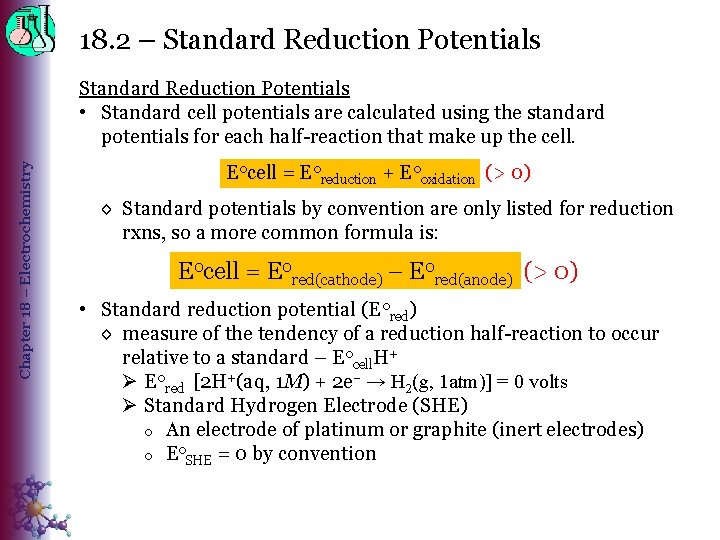
18. 2 – Standard Reduction Potentials Chapter 18 – Electrochemistry Standard Reduction Potentials • Standard cell potentials are calculated using the standard potentials for each half-reaction that make up the cell. E 0 cell = E 0 reduction + E 0 oxidation (> 0) ◊ Standard potentials by convention are only listed for reduction rxns, so a more common formula is: E 0 cell = E 0 red(cathode) – E 0 red(anode) (> 0) • Standard reduction potential (E 0 red) ◊ measure of the tendency of a reduction half-reaction to occur relative to a standard – E 0 cell. H+ Ø E 0 red [2 H+(aq, 1 M) + 2 e– → H 2(g, 1 atm)] = 0 volts Ø Standard Hydrogen Electrode (SHE) o An electrode of platinum or graphite (inert electrodes) o Eo. SHE = 0 by convention
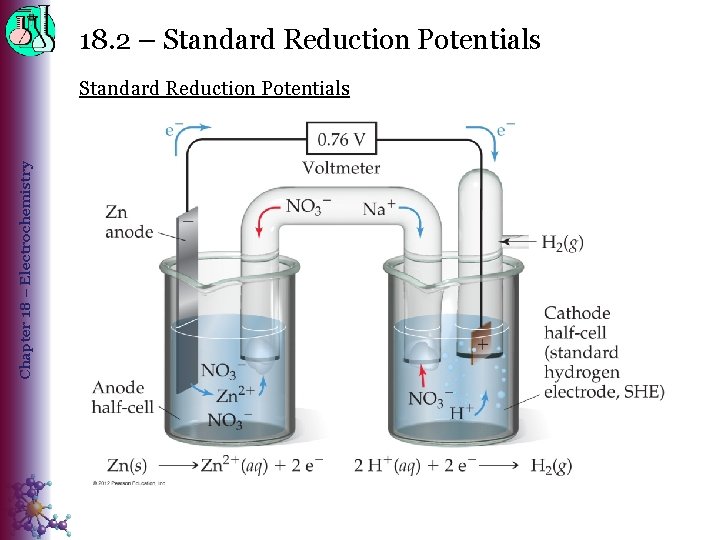
18. 2 – Standard Reduction Potentials Chapter 18 – Electrochemistry Standard Reduction Potentials
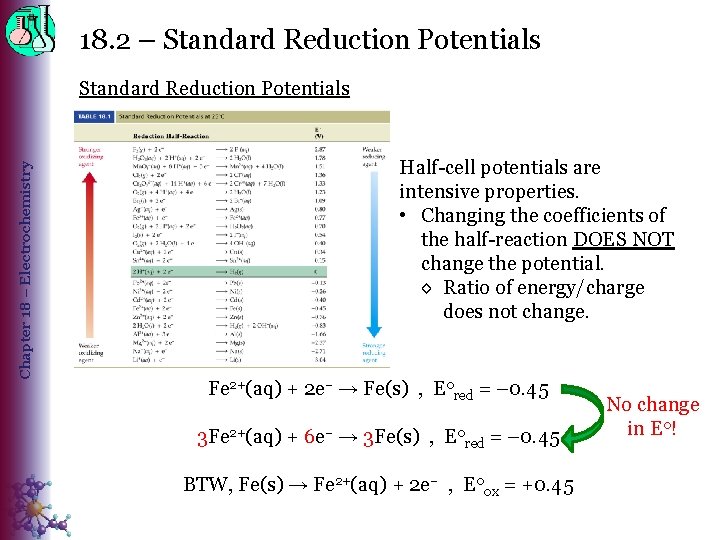
18. 2 – Standard Reduction Potentials Chapter 18 – Electrochemistry Standard Reduction Potentials Half-cell potentials are intensive properties. • Changing the coefficients of the half-reaction DOES NOT change the potential. ◊ Ratio of energy/charge does not change. Fe 2+(aq) + 2 e– → Fe(s) , E 0 red = – 0. 45 3 Fe 2+(aq) + 6 e– → 3 Fe(s) , E 0 red = – 0. 45 BTW, Fe(s) → Fe 2+(aq) + 2 e– , E 0 ox = +0. 45 No change in E 0!
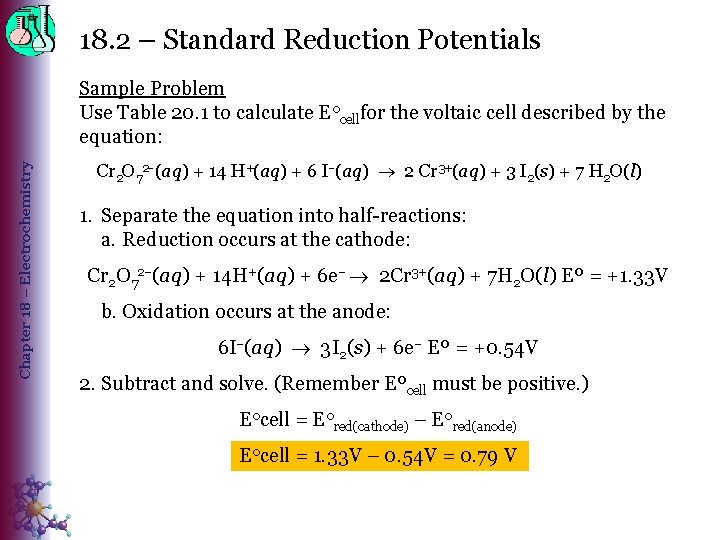
18. 2 – Standard Reduction Potentials Chapter 18 – Electrochemistry Sample Problem Use Table 20. 1 to calculate E 0 cellfor the voltaic cell described by the equation: Cr 2 O 72 (aq) + 14 H+(aq) + 6 I (aq) 2 Cr 3+(aq) + 3 I 2(s) + 7 H 2 O(l) 1. Separate the equation into half-reactions: a. Reduction occurs at the cathode: Cr 2 O 72 (aq) + 14 H+(aq) + 6 e– 2 Cr 3+(aq) + 7 H 2 O(l) Eº = +1. 33 V b. Oxidation occurs at the anode: 6 I (aq) 3 I 2(s) + 6 e– Eº = +0. 54 V 2. Subtract and solve. (Remember Eºcell must be positive. ) E 0 cell = E 0 red(cathode) – E 0 red(anode) E 0 cell = 1. 33 V – 0. 54 V = 0. 79 V
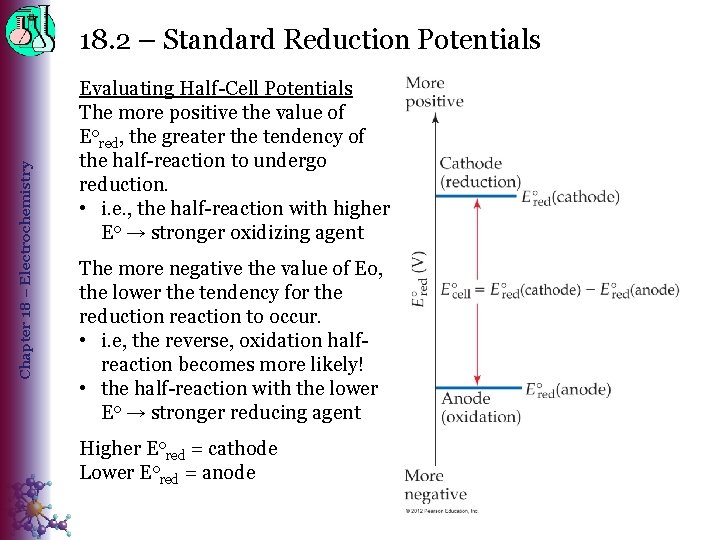
Chapter 18 – Electrochemistry 18. 2 – Standard Reduction Potentials Evaluating Half-Cell Potentials The more positive the value of Eored, the greater the tendency of the half-reaction to undergo reduction. • i. e. , the half-reaction with higher Eo → stronger oxidizing agent The more negative the value of Eo, the lower the tendency for the reduction reaction to occur. • i. e, the reverse, oxidation halfreaction becomes more likely! • the half-reaction with the lower Eo → stronger reducing agent Higher Eored = cathode Lower Eored = anode
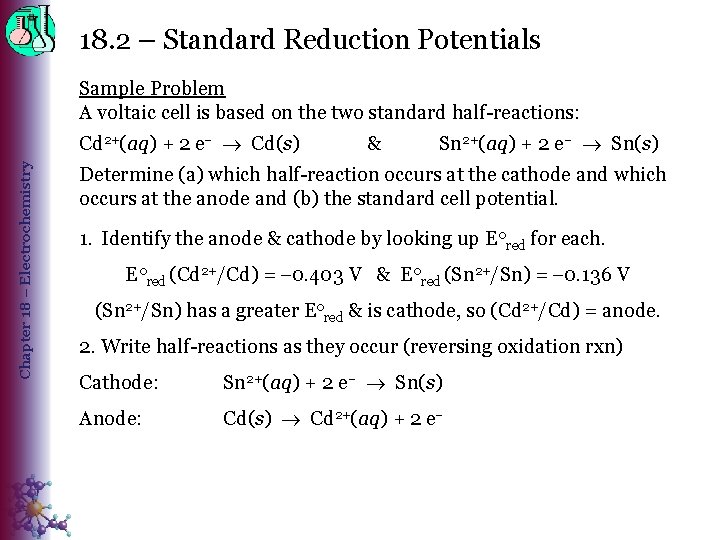
18. 2 – Standard Reduction Potentials Sample Problem A voltaic cell is based on the two standard half-reactions: Chapter 18 – Electrochemistry Cd 2+(aq) + 2 e Cd(s) & Sn 2+(aq) + 2 e– Sn(s) Determine (a) which half-reaction occurs at the cathode and which occurs at the anode and (b) the standard cell potential. 1. Identify the anode & cathode by looking up E 0 red for each. E 0 red (Cd 2+/Cd) = 0. 403 V & E 0 red (Sn 2+/Sn) = 0. 136 V (Sn 2+/Sn) has a greater Eored & is cathode, so (Cd 2+/Cd) = anode. 2. Write half-reactions as they occur (reversing oxidation rxn) Cathode: Sn 2+(aq) + 2 e– Sn(s) Anode: Cd(s) Cd 2+(aq) + 2 e
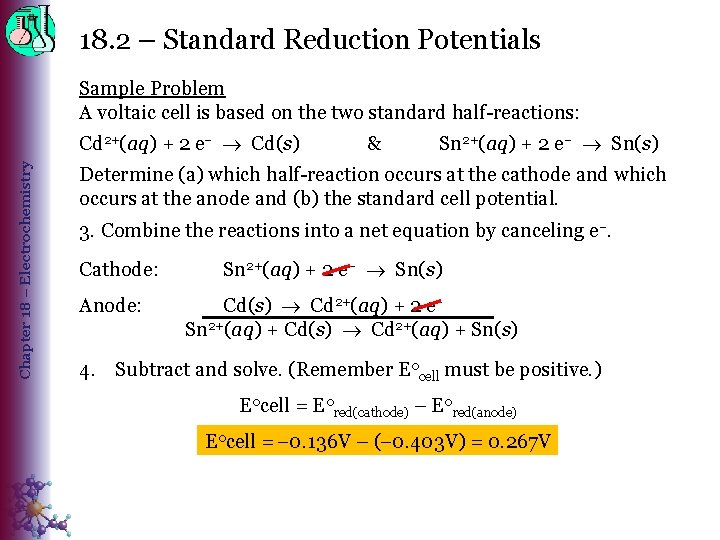
18. 2 – Standard Reduction Potentials Sample Problem A voltaic cell is based on the two standard half-reactions: Chapter 18 – Electrochemistry Cd 2+(aq) + 2 e Cd(s) & Sn 2+(aq) + 2 e– Sn(s) Determine (a) which half-reaction occurs at the cathode and which occurs at the anode and (b) the standard cell potential. 3. Combine the reactions into a net equation by canceling e–. Cathode: Anode: Sn 2+(aq) + 2 e– Sn(s) Cd(s) Cd 2+(aq) + 2 e Sn 2+(aq) + Cd(s) Cd 2+(aq) + Sn(s) 4. Subtract and solve. (Remember E 0 cell must be positive. ) E 0 cell = E 0 red(cathode) – E 0 red(anode) E 0 cell = 0. 136 V – ( 0. 403 V) = 0. 267 V
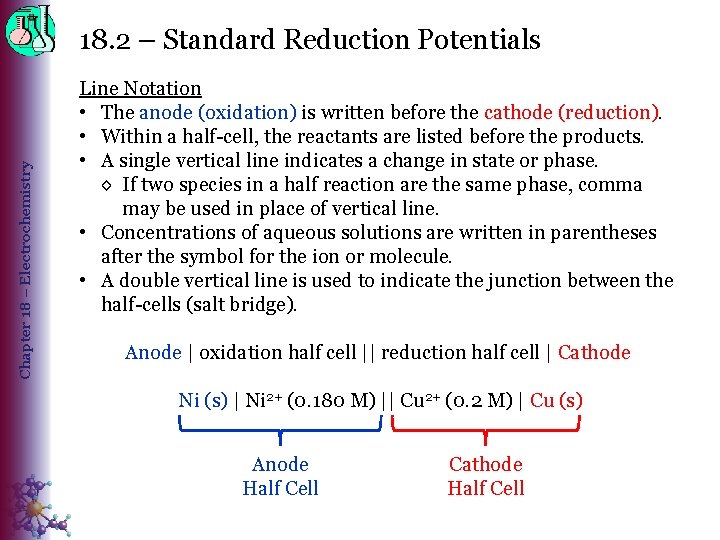
Chapter 18 – Electrochemistry 18. 2 – Standard Reduction Potentials Line Notation • The anode (oxidation) is written before the cathode (reduction). • Within a half-cell, the reactants are listed before the products. • A single vertical line indicates a change in state or phase. ◊ If two species in a half reaction are the same phase, comma may be used in place of vertical line. • Concentrations of aqueous solutions are written in parentheses after the symbol for the ion or molecule. • A double vertical line is used to indicate the junction between the half-cells (salt bridge). Anode | oxidation half cell || reduction half cell | Cathode Ni (s) | Ni 2+ (0. 180 M) || Cu 2+ (0. 2 M) | Cu (s) Anode Half Cell Cathode Half Cell
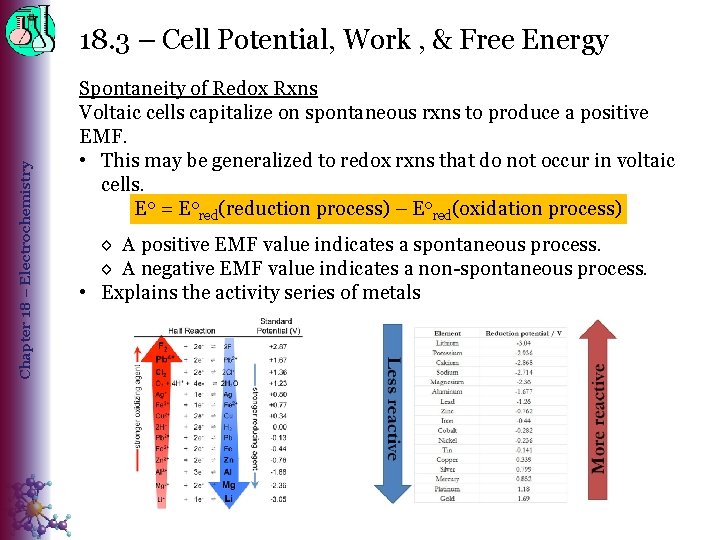
Chapter 18 – Electrochemistry 18. 3 – Cell Potential, Work , & Free Energy Spontaneity of Redox Rxns Voltaic cells capitalize on spontaneous rxns to produce a positive EMF. • This may be generalized to redox rxns that do not occur in voltaic cells. E 0 = E 0 red(reduction process) – Eored(oxidation process) ◊ A positive EMF value indicates a spontaneous process. ◊ A negative EMF value indicates a non-spontaneous process. • Explains the activity series of metals
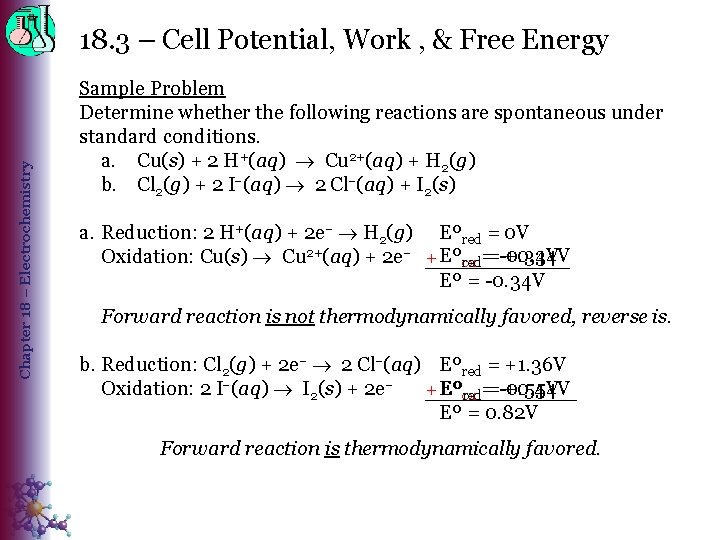
Chapter 18 – Electrochemistry 18. 3 – Cell Potential, Work , & Free Energy Sample Problem Determine whether the following reactions are spontaneous under standard conditions. a. Cu(s) + 2 H+(aq) Cu 2+(aq) + H 2(g) b. Cl 2(g) + 2 I (aq) 2 Cl (aq) + I 2(s) a. Reduction: 2 H+(aq) + 2 e– H 2(g) Eºred = 0 V =-0. 34 V +0. 34 V Oxidation: Cu(s) Cu 2+(aq) + 2 e– + Eºred ox = Eº = -0. 34 V Forward reaction is not thermodynamically favored, reverse is. b. Reduction: Cl 2(g) + 2 e– 2 Cl (aq) Eºred = +1. 36 V Oxidation: 2 I (aq) I 2(s) + 2 e– =-0. 54 V + Eºox red= Eº = 0. 82 V Forward reaction is thermodynamically favored.
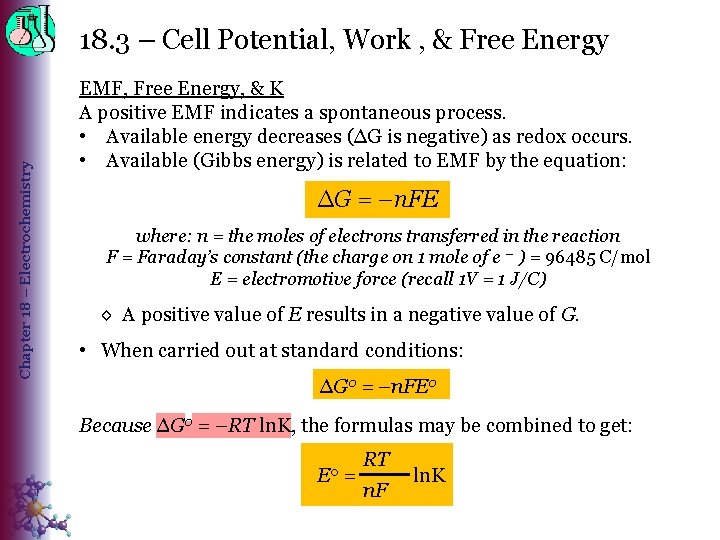
Chapter 18 – Electrochemistry 18. 3 – Cell Potential, Work , & Free Energy EMF, Free Energy, & K A positive EMF indicates a spontaneous process. • Available energy decreases (∆G is negative) as redox occurs. • Available (Gibbs energy) is related to EMF by the equation: ∆G = –n. FE where: n = the moles of electrons transferred in the reaction F = Faraday’s constant (the charge on 1 mole of e – ) = 96485 C/mol E = electromotive force (recall 1 V = 1 J/C) ◊ A positive value of E results in a negative value of G. • When carried out at standard conditions: ∆G 0 = –n. FE 0 Because ∆G 0 = –RT ln. K, the formulas may be combined to get: E 0 = RT n. F ln. K
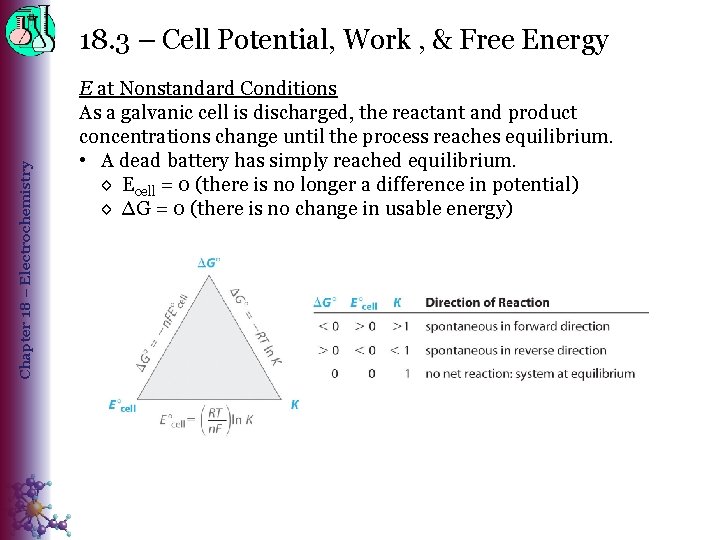
Chapter 18 – Electrochemistry 18. 3 – Cell Potential, Work , & Free Energy E at Nonstandard Conditions As a galvanic cell is discharged, the reactant and product concentrations change until the process reaches equilibrium. • A dead battery has simply reached equilibrium. ◊ Ecell = 0 (there is no longer a difference in potential) ◊ ∆G = 0 (there is no change in usable energy)
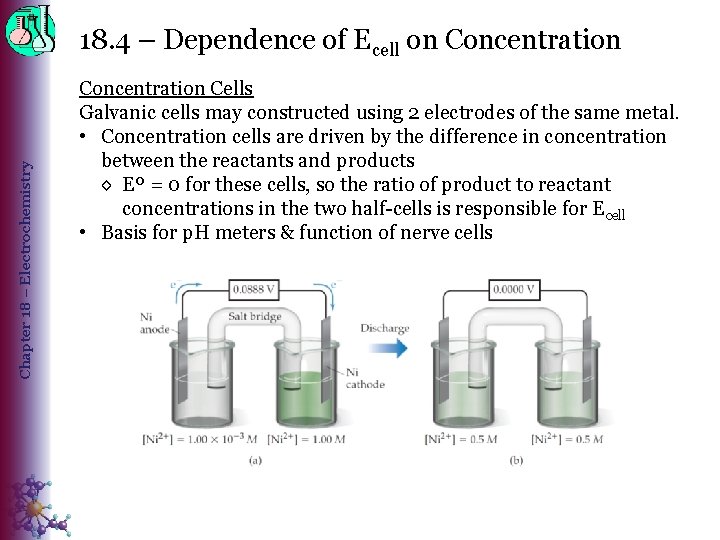
Chapter 18 – Electrochemistry 18. 4 – Dependence of Ecell on Concentration Cells Galvanic cells may constructed using 2 electrodes of the same metal. • Concentration cells are driven by the difference in concentration between the reactants and products ◊ Eº = 0 for these cells, so the ratio of product to reactant concentrations in the two half-cells is responsible for Ecell • Basis for p. H meters & function of nerve cells
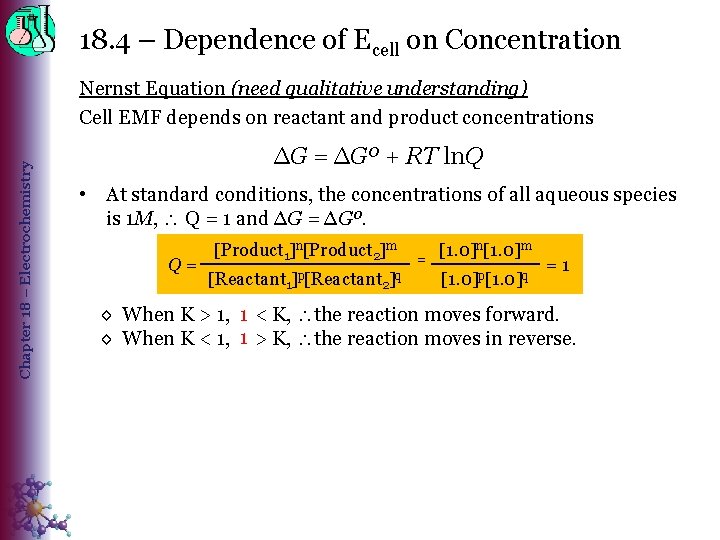
18. 4 – Dependence of Ecell on Concentration Chapter 18 – Electrochemistry Nernst Equation (need qualitative understanding) Cell EMF depends on reactant and product concentrations ∆G = ∆Gº + RT ln. Q • At standard conditions, the concentrations of all aqueous species is 1 M, Q = 1 and ∆G = ∆Gº. Q= [Product 1]n[Product 2]m [Reactant 1 ]p[Reactant q 2] = [1. 0]n[1. 0]m [1. 0]p[1. 0]q =1 1 < K, the reaction moves forward. ◊ When K > 1, Q 1 > K, the reaction moves in reverse. ◊ When K < 1, Q
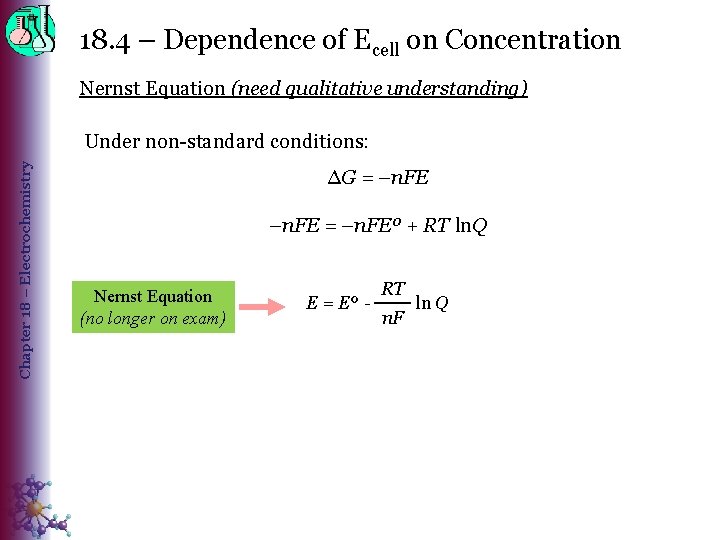
18. 4 – Dependence of Ecell on Concentration Nernst Equation (need qualitative understanding) Chapter 18 – Electrochemistry Under non-standard conditions: ∆G = –n. FEº + RT ln. Q Nernst Equation (no longer on exam) E = Eº - RT n. F ln Q
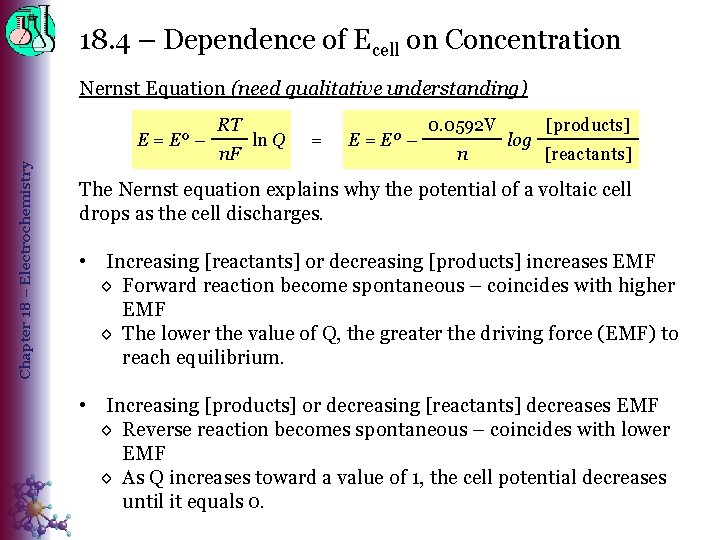
18. 4 – Dependence of Ecell on Concentration Nernst Equation (need qualitative understanding) Chapter 18 – Electrochemistry E = Eº – RT n. F ln Q = Eº – 0. 0592 V n log [products] [reactants] The Nernst equation explains why the potential of a voltaic cell drops as the cell discharges. • Increasing [reactants] or decreasing [products] increases EMF ◊ Forward reaction become spontaneous – coincides with higher EMF ◊ The lower the value of Q, the greater the driving force (EMF) to reach equilibrium. • Increasing [products] or decreasing [reactants] decreases EMF ◊ Reverse reaction becomes spontaneous – coincides with lower EMF ◊ As Q increases toward a value of 1, the cell potential decreases until it equals 0.
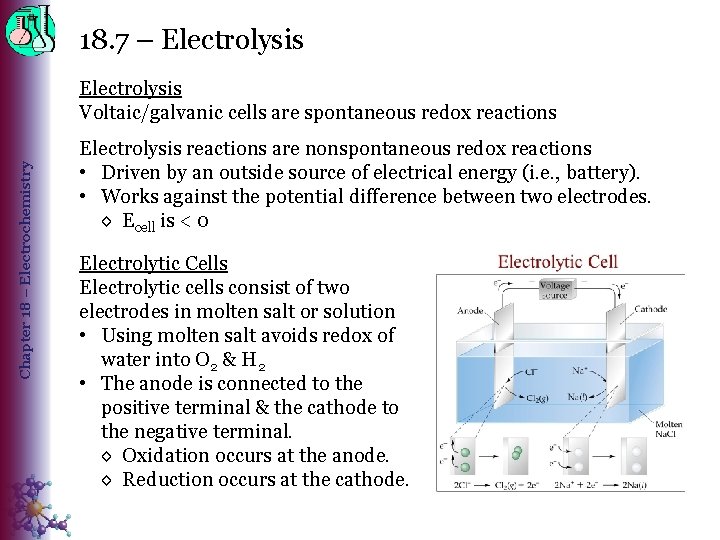
18. 7 – Electrolysis Chapter 18 – Electrochemistry Electrolysis Voltaic/galvanic cells are spontaneous redox reactions Electrolysis reactions are nonspontaneous redox reactions • Driven by an outside source of electrical energy (i. e. , battery). • Works against the potential difference between two electrodes. ◊ Ecell is < 0 Electrolytic Cells Electrolytic cells consist of two electrodes in molten salt or solution • Using molten salt avoids redox of water into O 2 & H 2 • The anode is connected to the positive terminal & the cathode to the negative terminal. ◊ Oxidation occurs at the anode. ◊ Reduction occurs at the cathode.
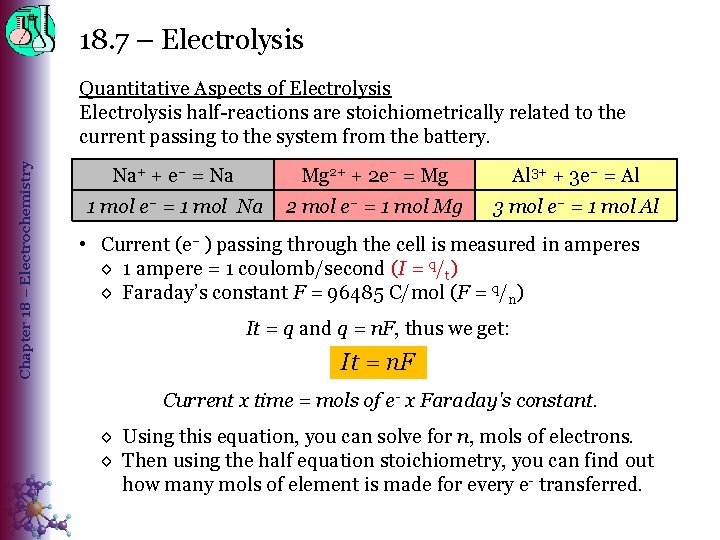
18. 7 – Electrolysis Chapter 18 – Electrochemistry Quantitative Aspects of Electrolysis half-reactions are stoichiometrically related to the current passing to the system from the battery. Na+ + e– = Na Mg 2+ + 2 e– = Mg Al 3+ + 3 e– = Al 1 mol e– = 1 mol Na 2 mol e– = 1 mol Mg 3 mol e– = 1 mol Al • Current (e– ) passing through the cell is measured in amperes ◊ 1 ampere = 1 coulomb/second (I = q/t) ◊ Faraday’s constant F = 96485 C/mol (F = q/n) It = q and q = n. F, thus we get: It = n. F Current x time = mols of e- x Faraday's constant. ◊ Using this equation, you can solve for n, mols of electrons. ◊ Then using the half equation stoichiometry, you can find out how many mols of element is made for every e- transferred.
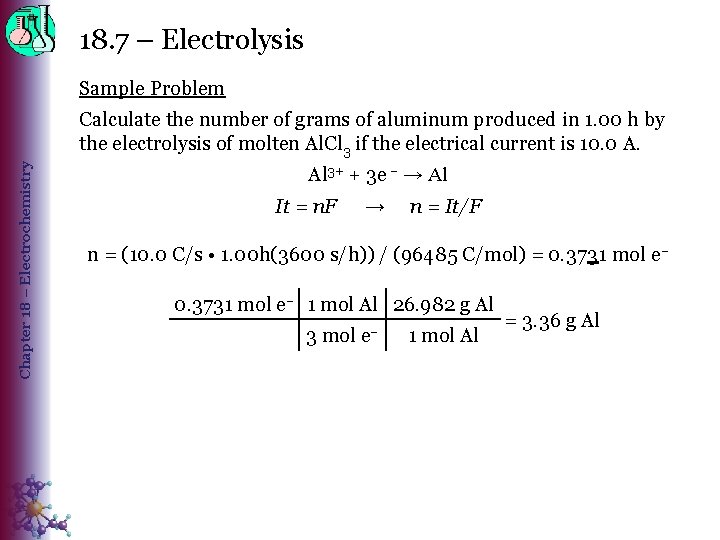
18. 7 – Electrolysis Sample Problem Chapter 18 – Electrochemistry Calculate the number of grams of aluminum produced in 1. 00 h by the electrolysis of molten Al. Cl 3 if the electrical current is 10. 0 A. Al 3+ + 3 e – → Al It = n. F → n = It/F n = (10. 0 C/s • 1. 00 h(3600 s/h)) / (96485 C/mol) = 0. 3731 mol e– 1 mol Al 26. 982 g Al 3 mol e– 1 mol Al = 3. 36 g Al
Example of redox reaction
Chapter 19 redox reactions answers
Redox rules
How do oxidation numbers work
Reactions that produce gas
Log sig fig
What is a half reaction
Examples of redox reaction
Leo and ger
Complex redox reactions
Balancing redox reactions in acid
Balancing redox reactions
Activity series oxidation
How do you balance a chemical equation
Writing half reactions
Redox reaction
Redox reactions ncea level 2
Chemsheets combining half equations
How redox reactions work
Accomplish the concept map on redox reaction
Electrolysis khan academy
What are spectator ions
Voltaic cell components
Redox table
Section 2 classifying chemical reactions worksheet answers
Section 2 classifying chemical reactions worksheet answers
Chemical reactions section 3 reactions in aqueous solutions
Unit 5 chemical reactions answers
Ap chemistry chapter 18 electrochemistry test
Chapter 20 review electrochemistry
Ap chem electrochemistry review
Cell chapter 21
Difference between galvanic cell and electrolytic cell
Chapter 20 electrochemistry
Chapter 10 chapter assessment chemical reactions answers