The Atomic Force Microscope as versatile tool in
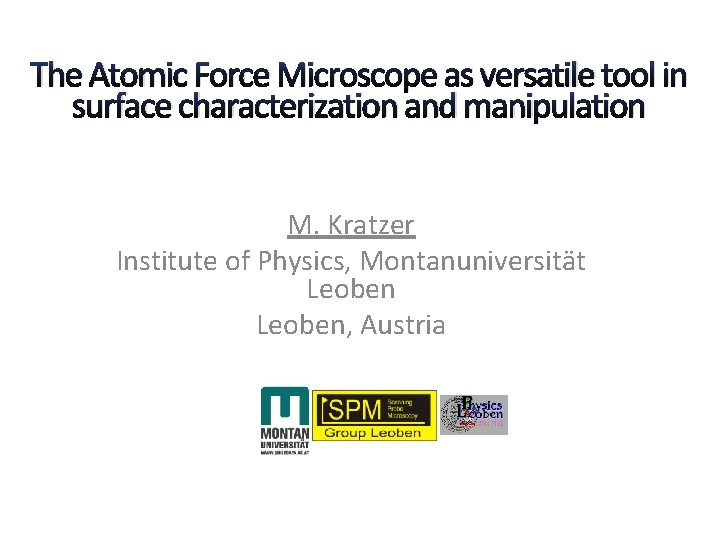
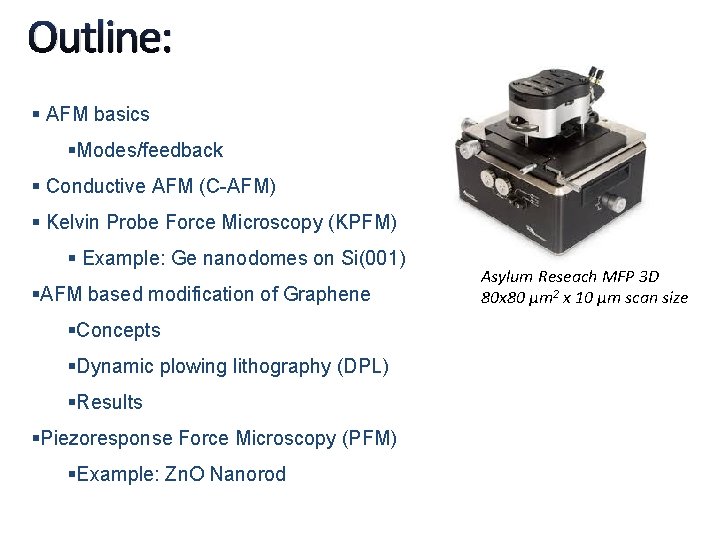
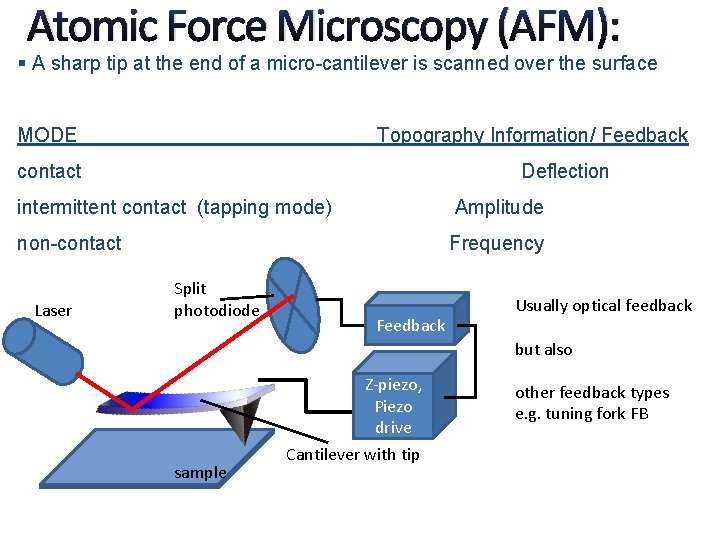
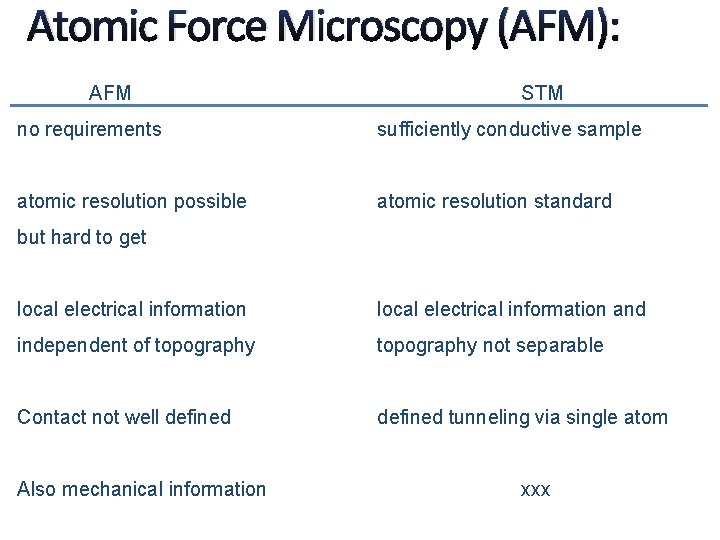
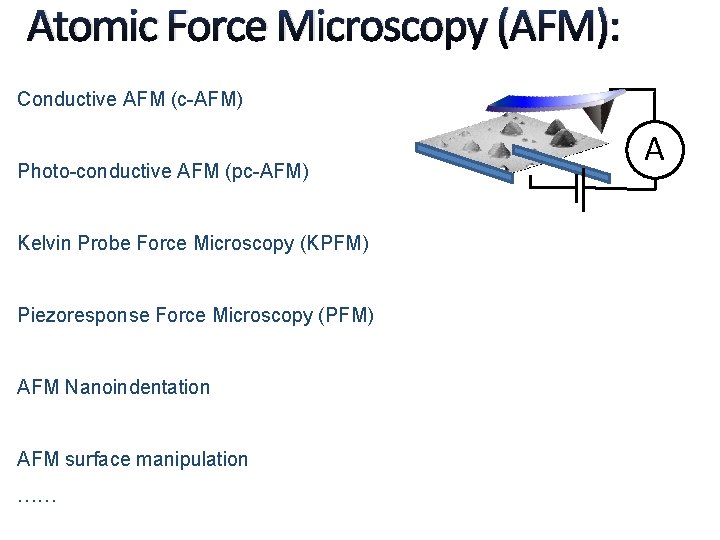
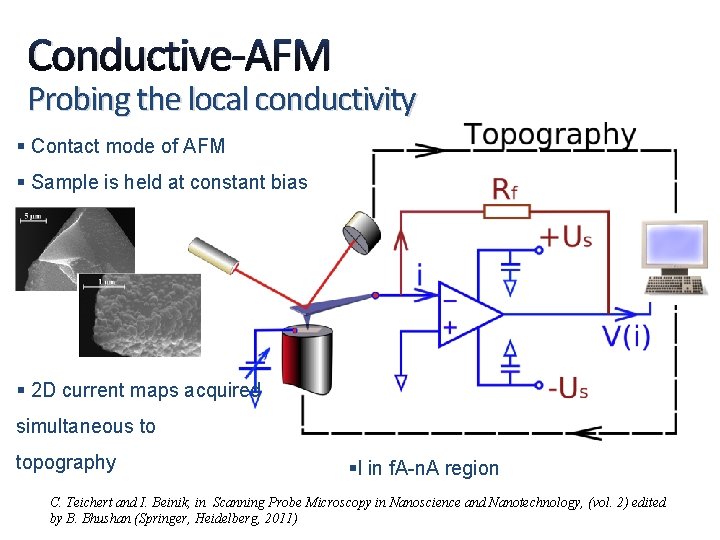
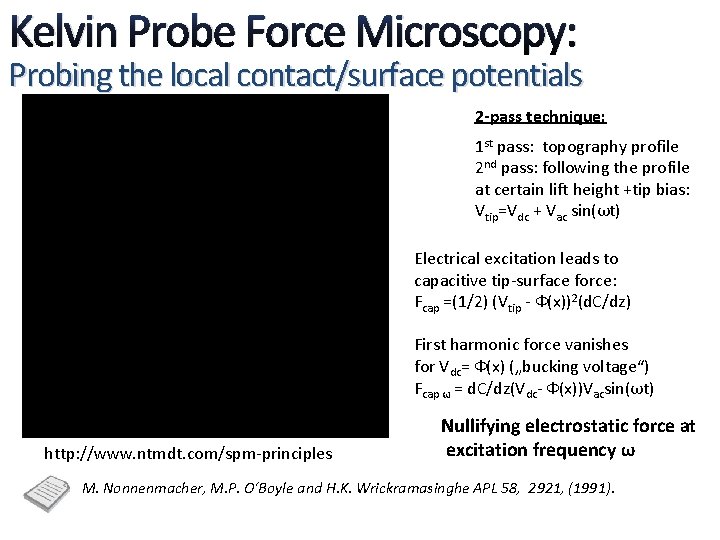
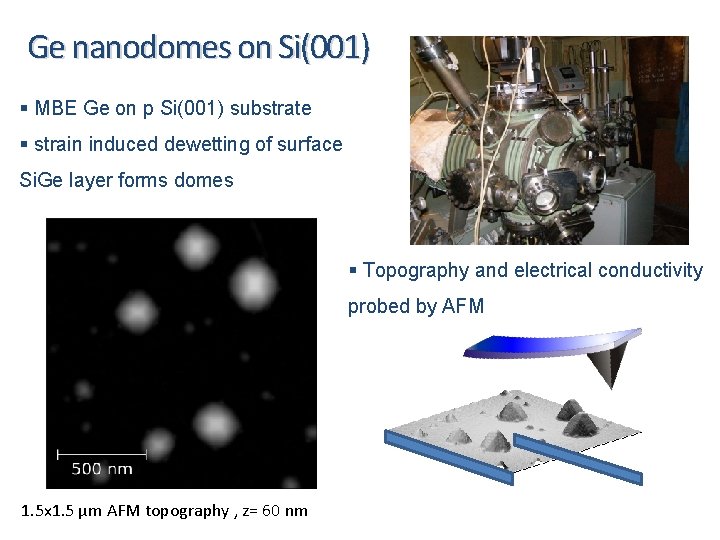
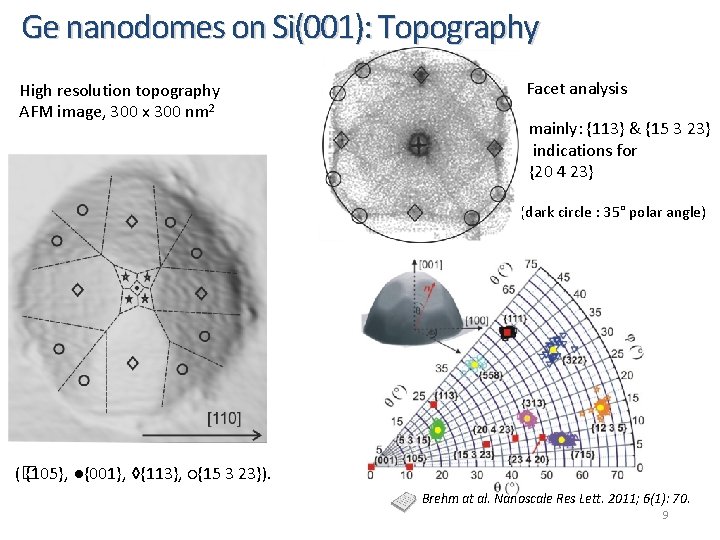
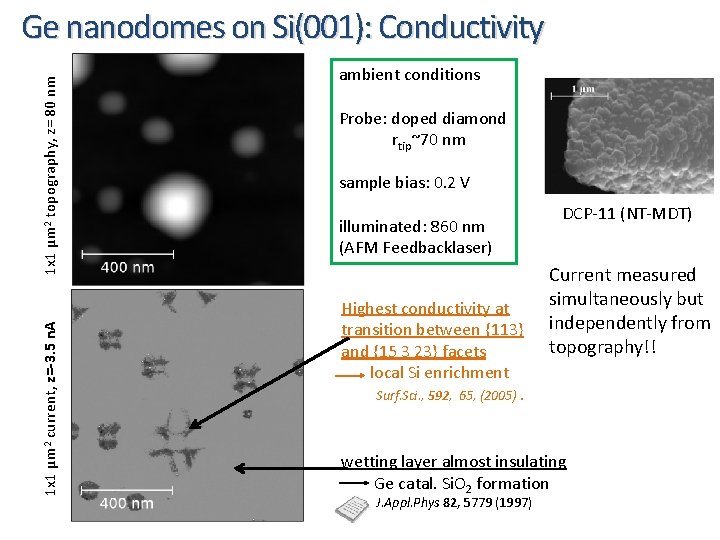
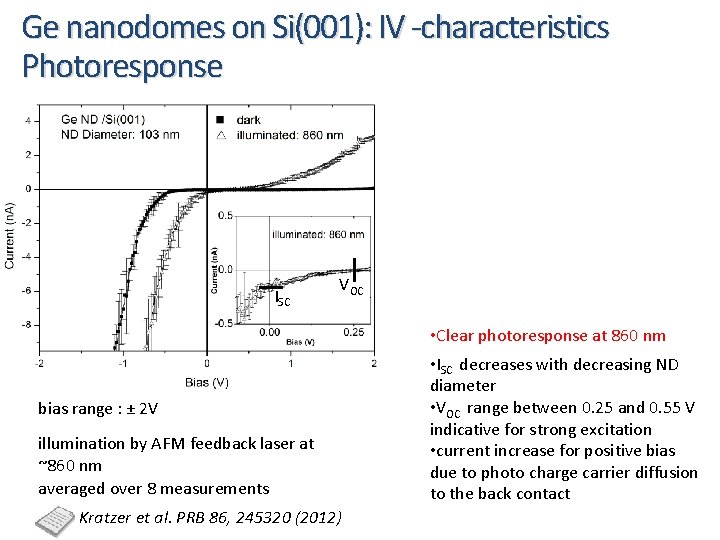
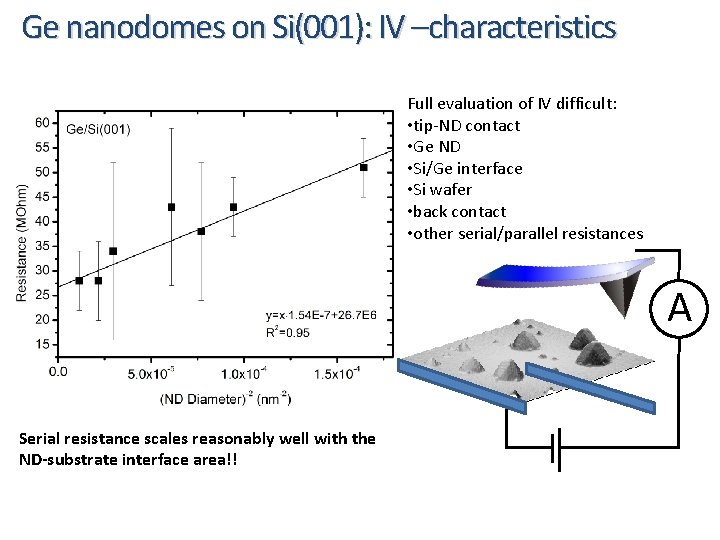
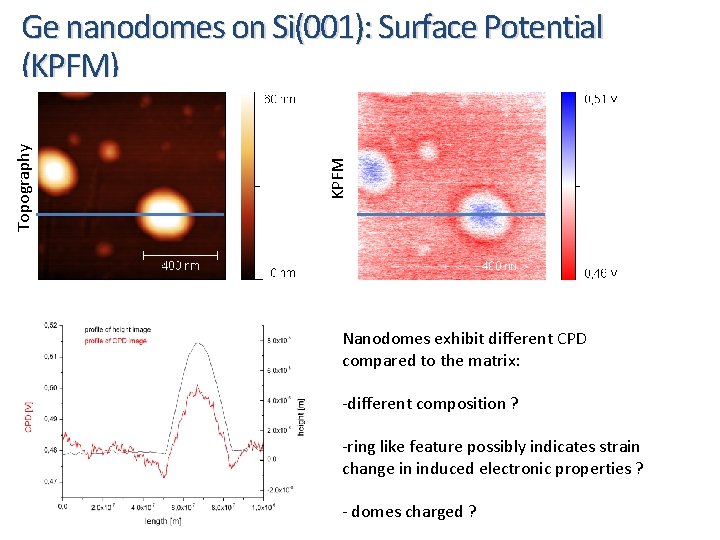
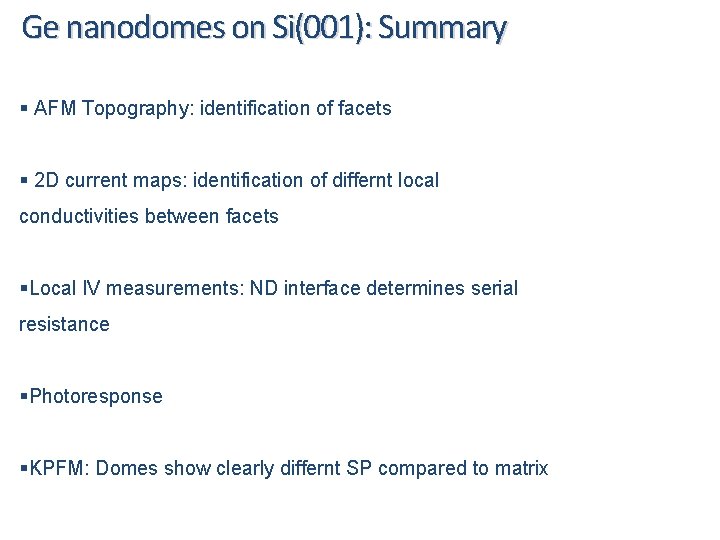
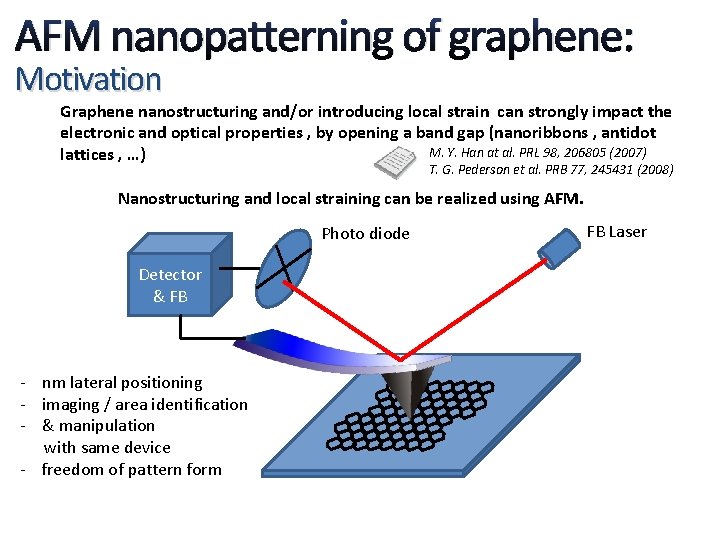
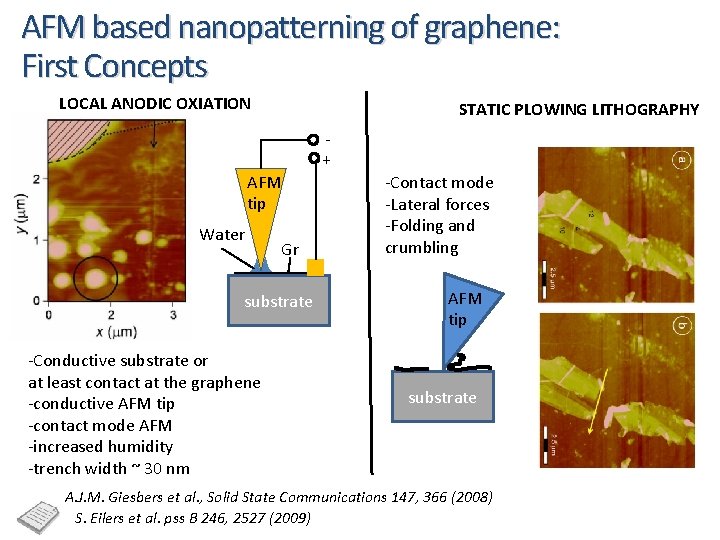
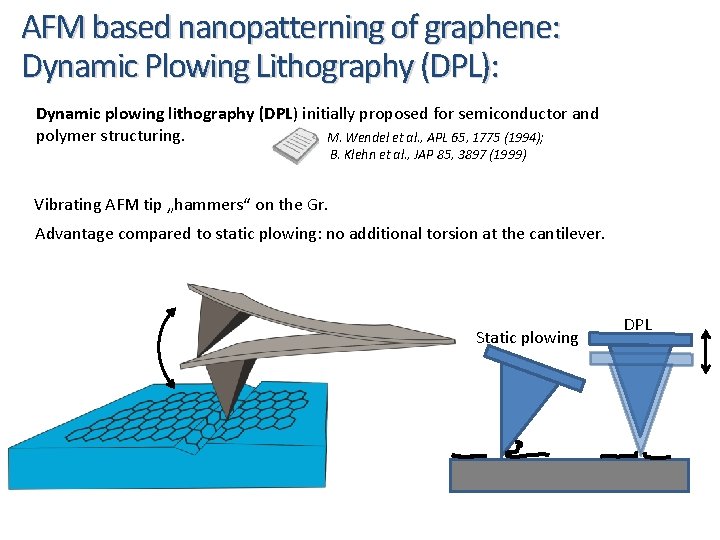
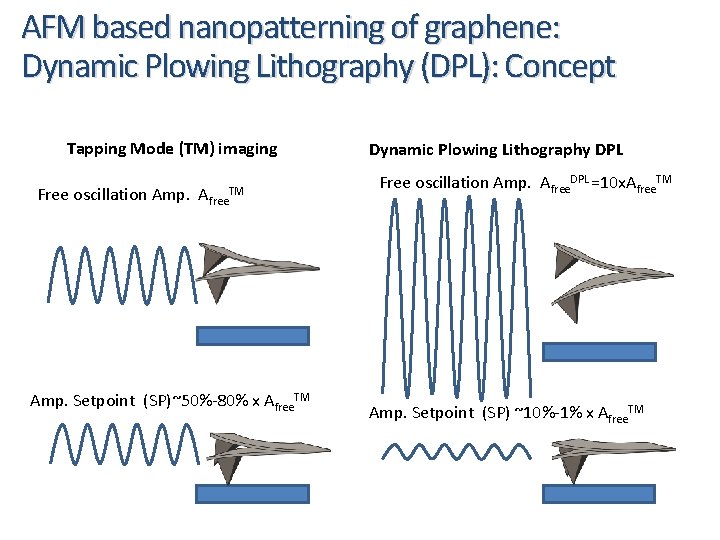
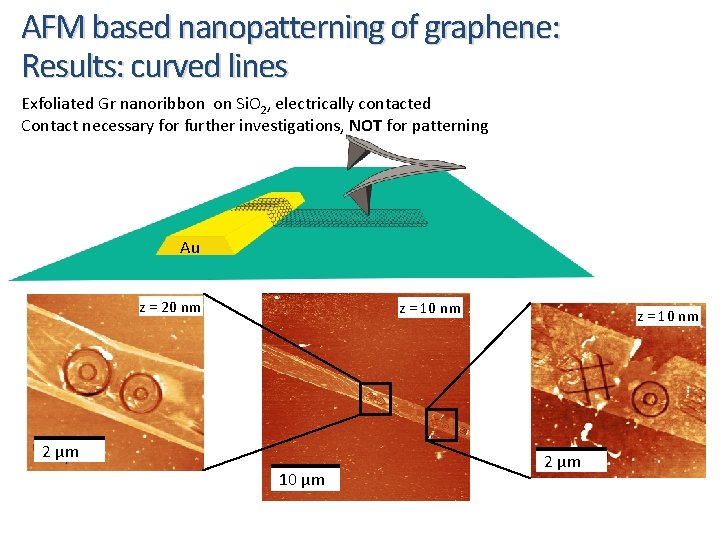
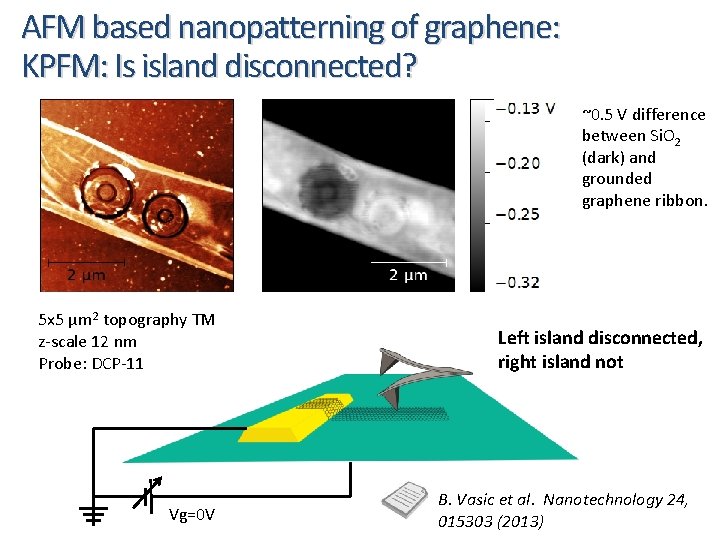
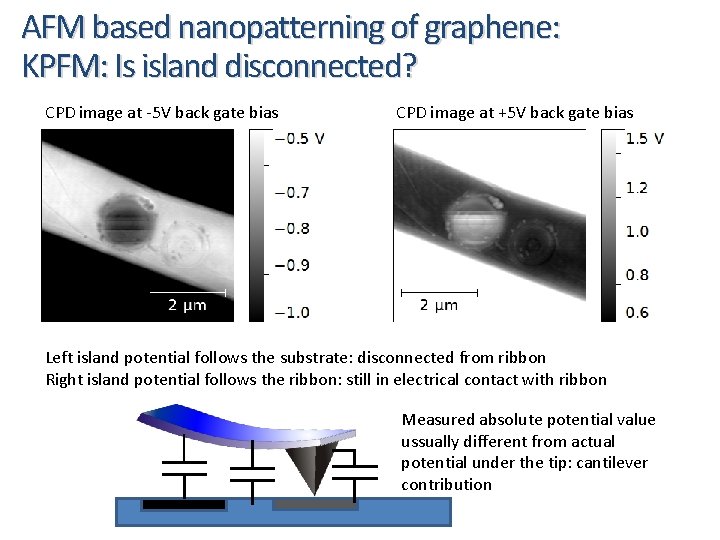
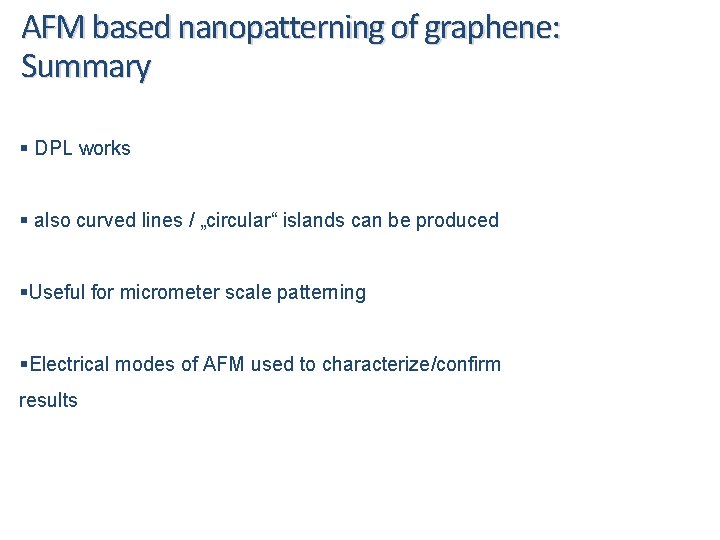
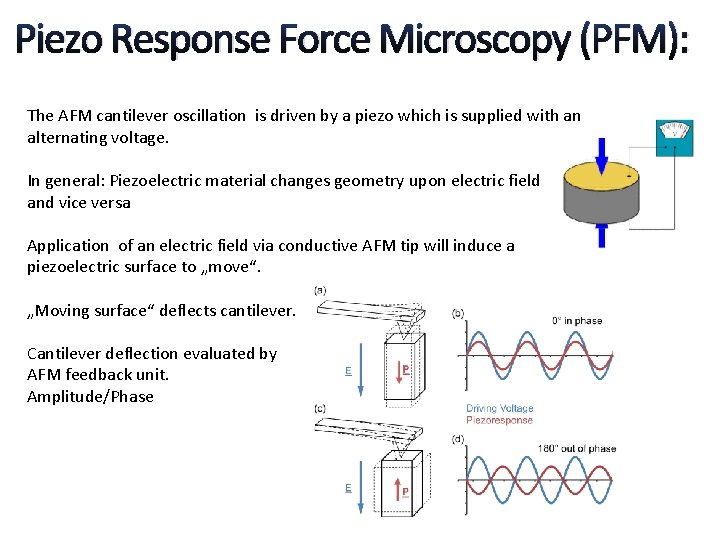
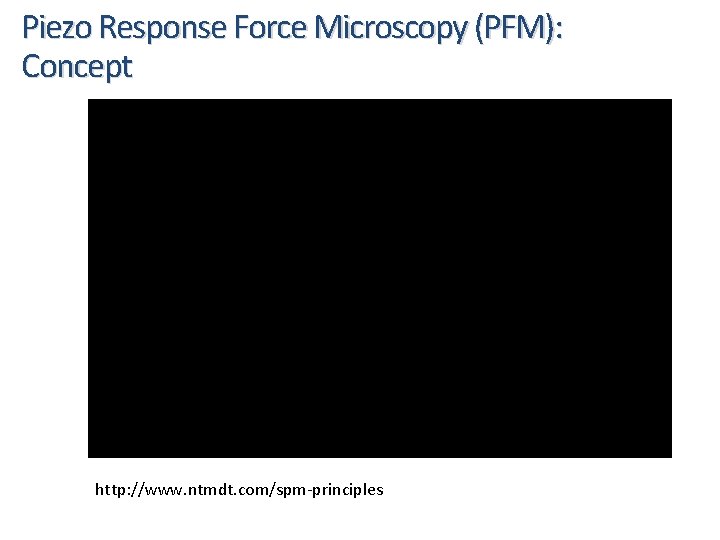
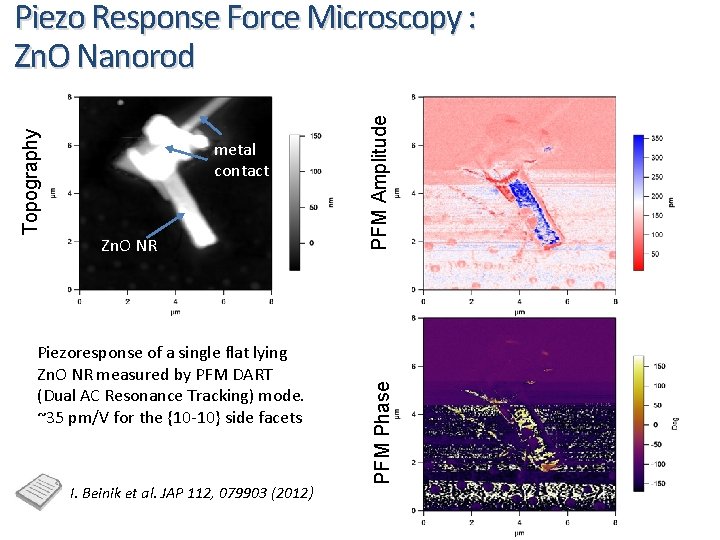
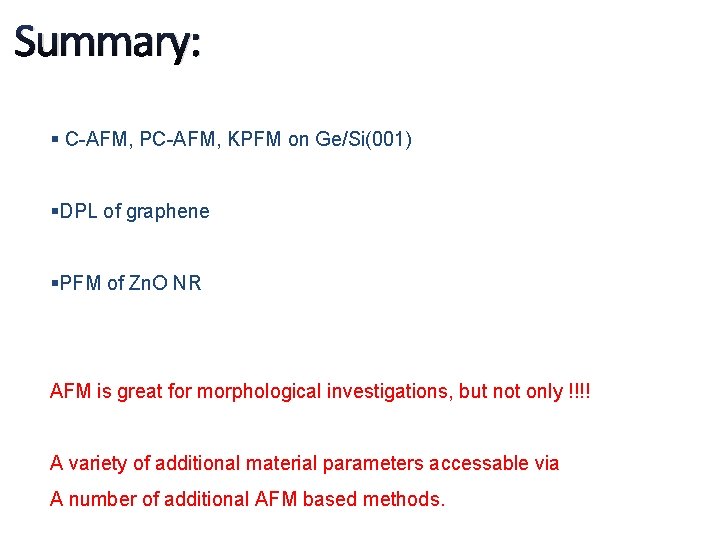
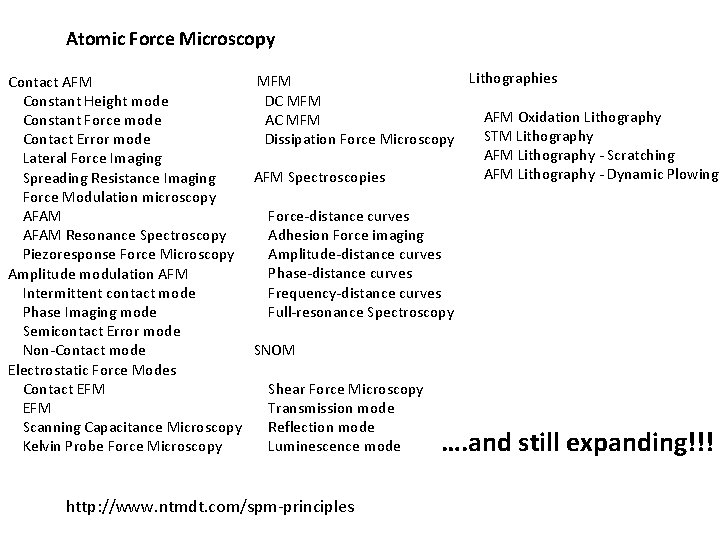
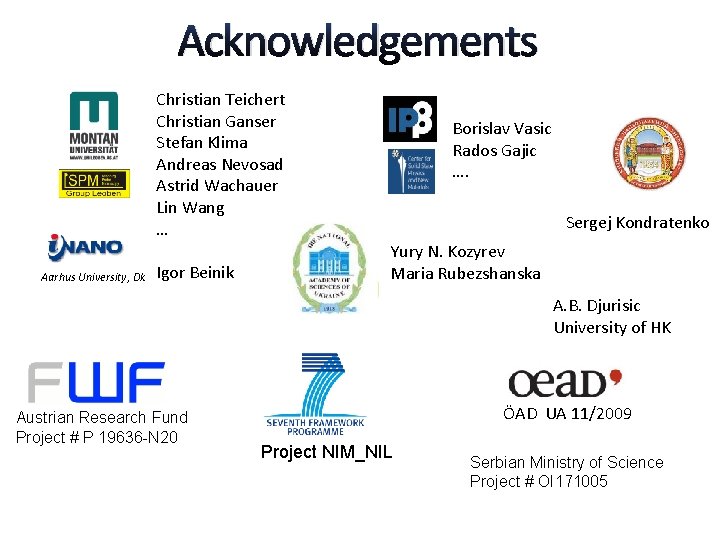
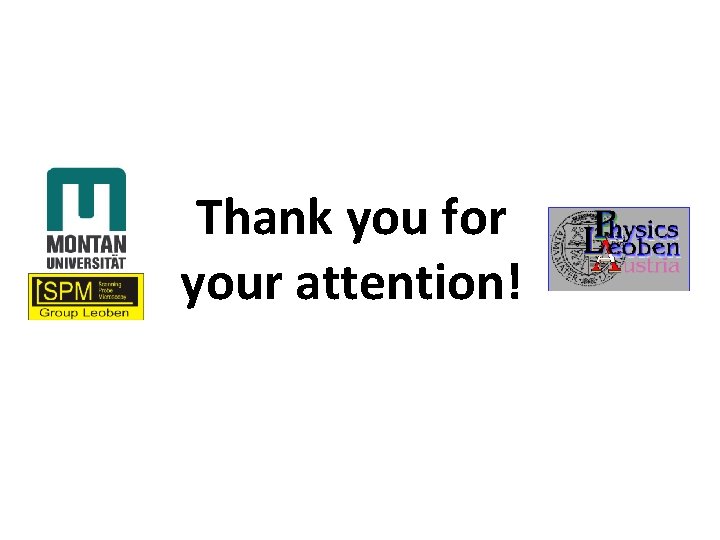
- Slides: 29
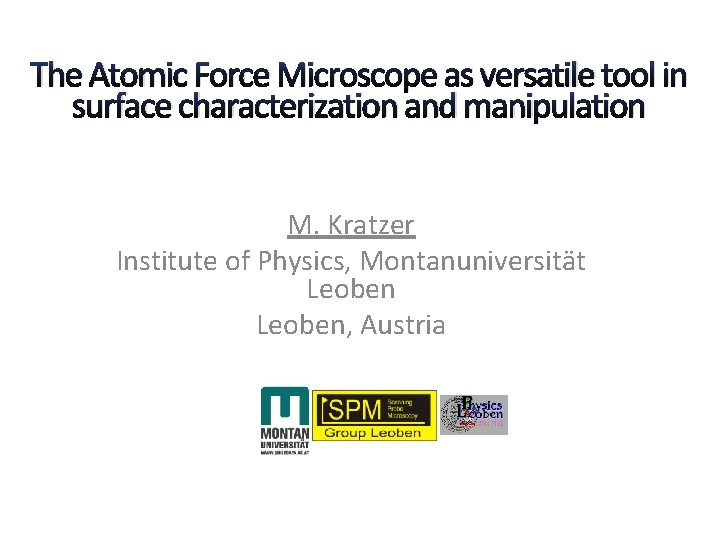
The Atomic Force Microscope as versatile tool in surface characterization and manipulation M. Kratzer Institute of Physics, Montanuniversität Leoben, Austria
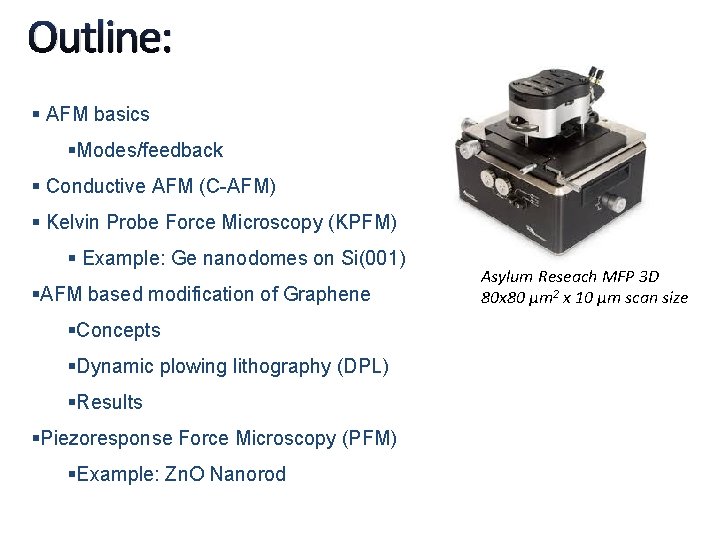
Outline: § AFM basics §Modes/feedback § Conductive AFM (C-AFM) § Kelvin Probe Force Microscopy (KPFM) § Example: Ge nanodomes on Si(001) §AFM based modification of Graphene §Concepts §Dynamic plowing lithography (DPL) §Results §Piezoresponse Force Microscopy (PFM) §Example: Zn. O Nanorod Asylum Reseach MFP 3 D 80 x 80 µm 2 x 10 µm scan size
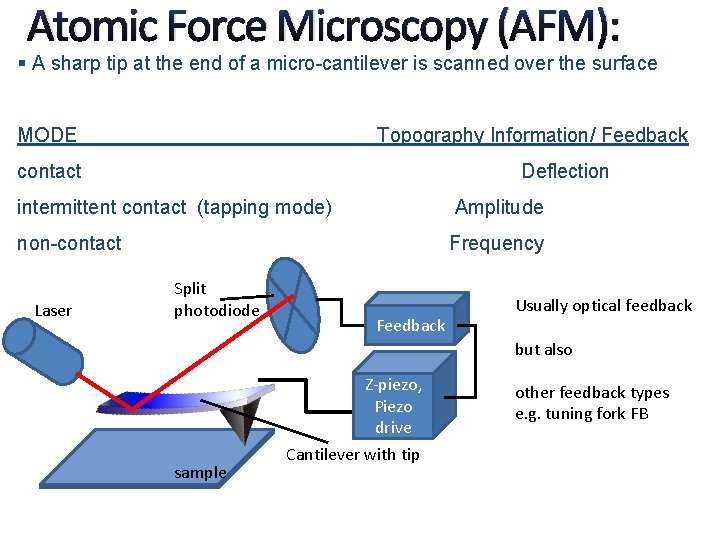
Atomic Force Microscopy (AFM): § A sharp tip at the end of a micro-cantilever is scanned over the surface MODE Topography Information/ Feedback contact Deflection intermittent contact (tapping mode) Amplitude non-contact Frequency Laser Split photodiode Feedback Usually optical feedback but also sample Z-piezo, Piezo drive Cantilever with tip other feedback types e. g. tuning fork FB
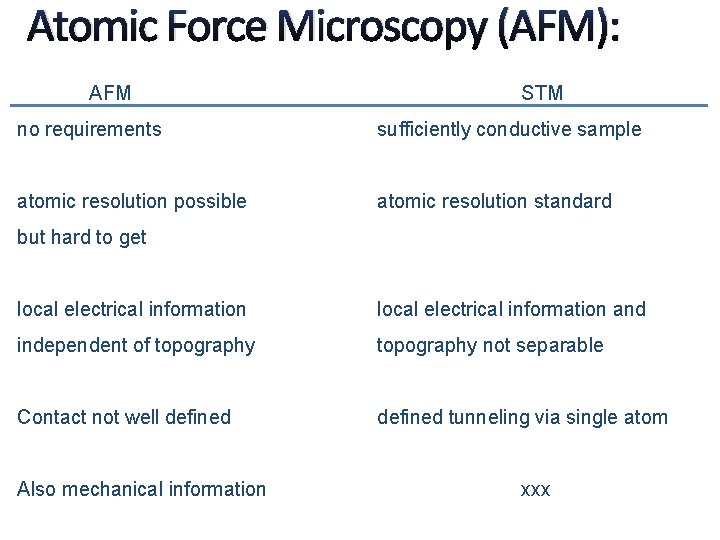
Atomic Force Microscopy (AFM): AFM STM no requirements sufficiently conductive sample atomic resolution possible atomic resolution standard but hard to get local electrical information and independent of topography not separable Contact not well defined tunneling via single atom Also mechanical information xxx
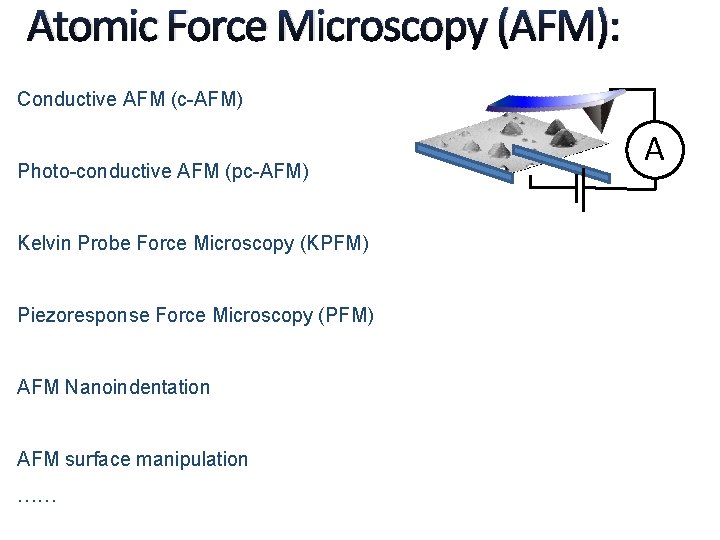
Atomic Force Microscopy (AFM): Photo-conductive AFM (pc-AFM) Kelvin Probe Force Microscopy (KPFM) Piezoresponse Force Microscopy (PFM) AFM Nanoindentation AFM surface manipulation …… Conductive AFM (c-AFM) A
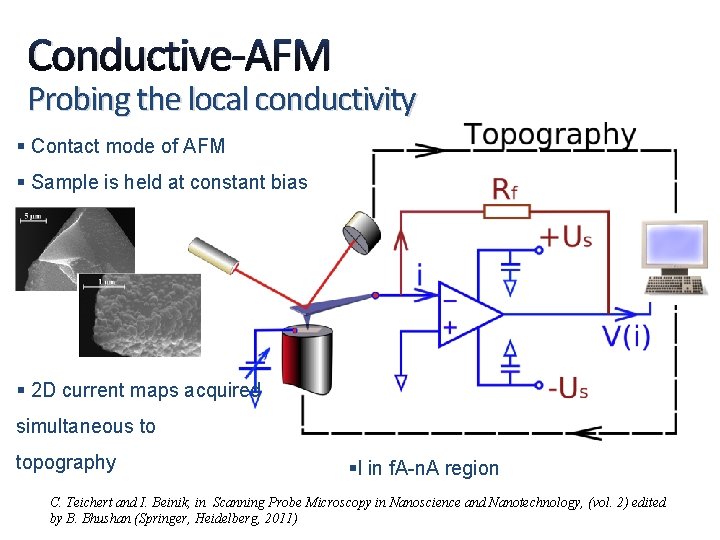
Conductive-AFM Probing the local conductivity § Contact mode of AFM § Sample is held at constant bias § 2 D current maps acquired simultaneous to topography §I in f. A-n. A region C. Teichert and I. Beinik, in Scanning Probe Microscopy in Nanoscience and Nanotechnology, (vol. 2) edited by B. Bhushan (Springer, Heidelberg, 2011)
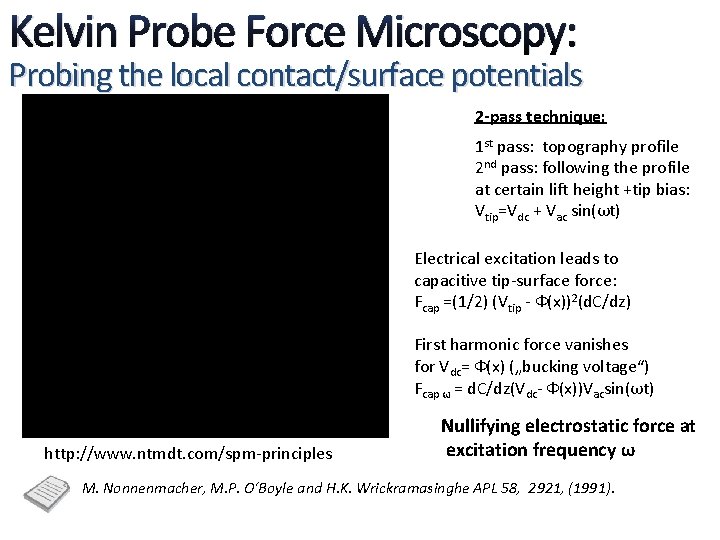
Kelvin Probe Force Microscopy: Probing the local contact/surface potentials 2 -pass technique: 1 st pass: topography profile 2 nd pass: following the profile at certain lift height +tip bias: Vtip=Vdc + Vac sin(ωt) Electrical excitation leads to capacitive tip-surface force: Fcap =(1/2) (Vtip - Ф(x))2(d. C/dz) First harmonic force vanishes for Vdc= Ф(x) („bucking voltage“) Fcap ω = d. C/dz(Vdc- Ф(x))Vacsin(ωt) http: //www. ntmdt. com/spm-principles Nullifying electrostatic force at excitation frequency ω M. Nonnenmacher, M. P. O‘Boyle and H. K. Wrickramasinghe APL 58, 2921, (1991).
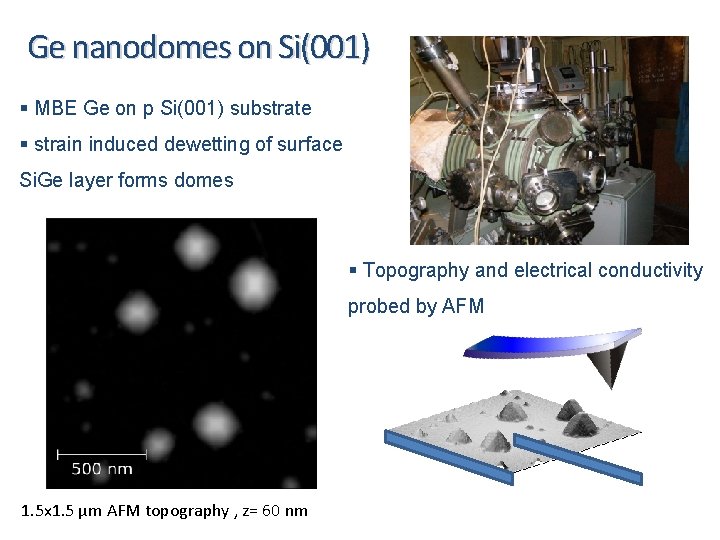
Ge nanodomes on Si(001) § MBE Ge on p Si(001) substrate § strain induced dewetting of surface Si. Ge layer forms domes § Topography and electrical conductivity 1. 5 x 1. 5 µm AFM topography , z= 60 nm probed by AFM
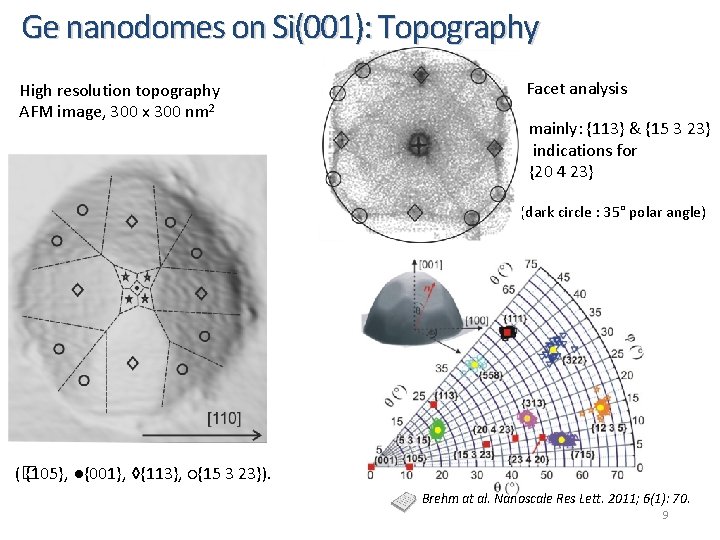
Ge nanodomes on Si(001): Topography High resolution topography AFM image, 300 x 300 nm 2 {15 3 23} Facet analysis mainly: {113} & {15 3 23} indications for {20 4 23} (dark circle : 35° polar angle) (� {105}, ●{001}, ◊{113}, ○{15 3 23}). Brehm at al. Nanoscale Res Lett. 2011; 6(1): 70. 9
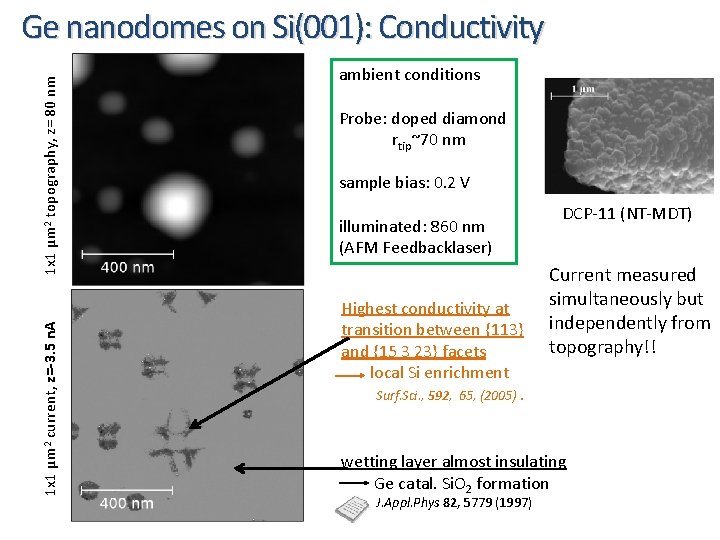
1 x 1 µm 2 current, z=-3. 5 n. A 1 x 1 µm 2 topography, z= 80 nm Ge nanodomes on Si(001): Conductivity ambient conditions Probe: doped diamond rtip~70 nm sample bias: 0. 2 V illuminated: 860 nm (AFM Feedbacklaser) Highest conductivity at transition between {113} and {15 3 23} facets local Si enrichment Surf. Sci. , 592, 65, (2005). DCP-11 (NT-MDT) Current measured simultaneously but independently from topography!! wetting layer almost insulating Ge catal. Si. O 2 formation J. Appl. Phys 82, 5779 (1997)
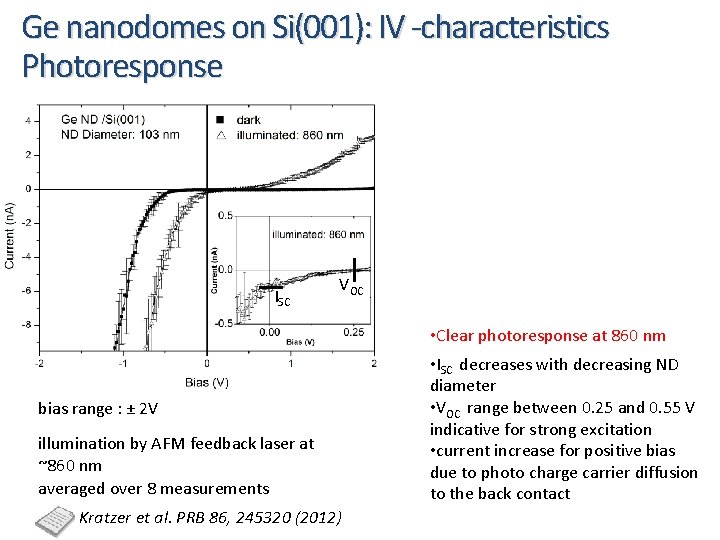
Ge nanodomes on Si(001): IV -characteristics Photoresponse ISC VOC • Clear photoresponse at 860 nm bias range : ± 2 V illumination by AFM feedback laser at ~860 nm averaged over 8 measurements Kratzer et al. PRB 86, 245320 (2012) • ISC decreases with decreasing ND diameter • VOC range between 0. 25 and 0. 55 V indicative for strong excitation • current increase for positive bias due to photo charge carrier diffusion to the back contact
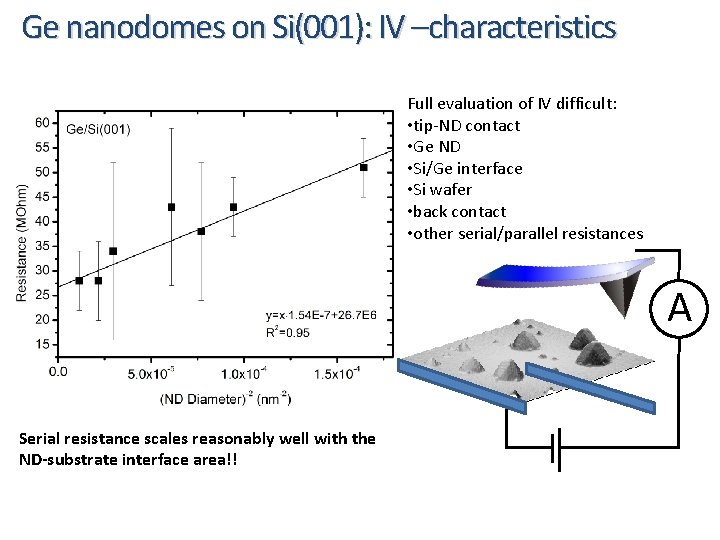
Ge nanodomes on Si(001): IV –characteristics Full evaluation of IV difficult: • tip-ND contact • Ge ND • Si/Ge interface • Si wafer • back contact • other serial/parallel resistances A Serial resistance scales reasonably well with the ND-substrate interface area!!
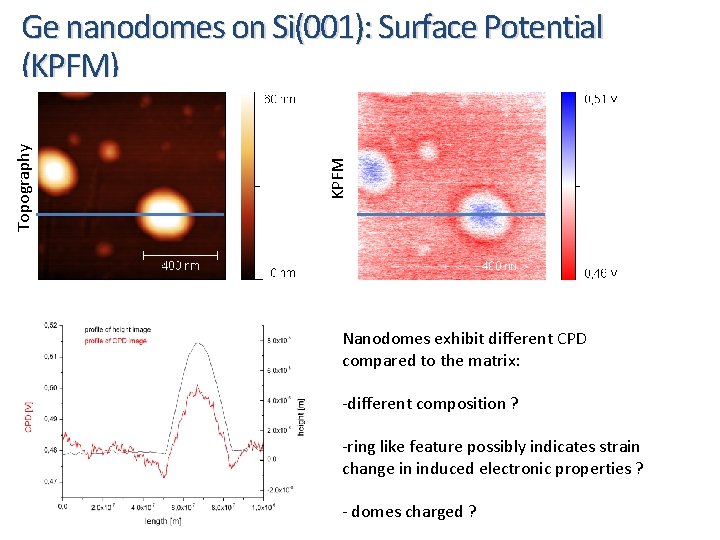
KPFM Topography Ge nanodomes on Si(001): Surface Potential (KPFM) Nanodomes exhibit different CPD compared to the matrix: -different composition ? -ring like feature possibly indicates strain change in induced electronic properties ? - domes charged ?
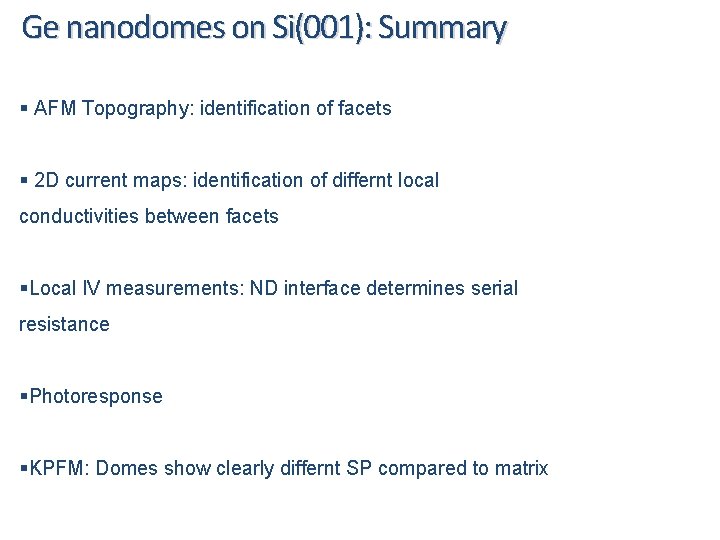
Ge nanodomes on Si(001): Summary § AFM Topography: identification of facets § 2 D current maps: identification of differnt local conductivities between facets §Local IV measurements: ND interface determines serial resistance §Photoresponse §KPFM: Domes show clearly differnt SP compared to matrix
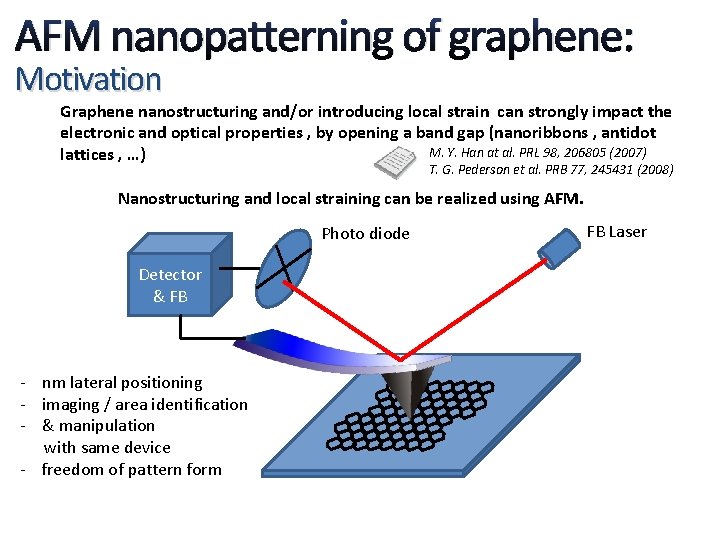
AFM nanopatterning of graphene: Motivation Graphene nanostructuring and/or introducing local strain can strongly impact the electronic and optical properties , by opening a band gap (nanoribbons , antidot M. Y. Han at al. PRL 98, 206805 (2007) lattices , …) T. G. Pederson et al. PRB 77, 245431 (2008) Nanostructuring and local straining can be realized using AFM. Photo diode Detector & FB - nm lateral positioning - imaging / area identification - & manipulation with same device - freedom of pattern form FB Laser
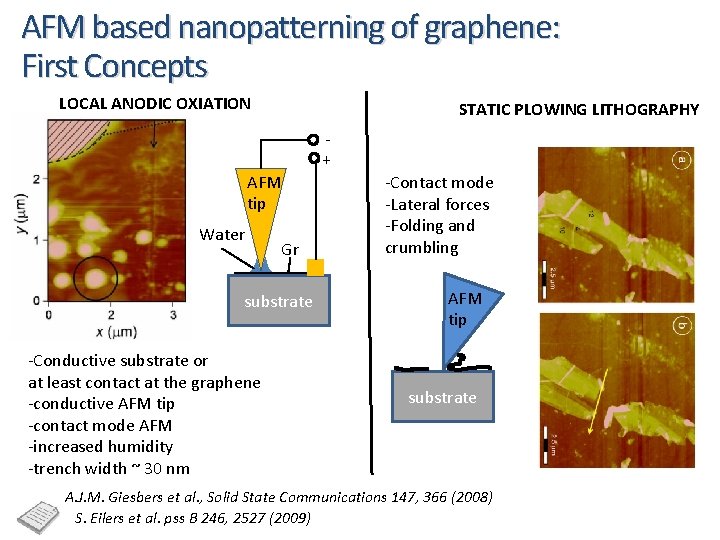
AFM based nanopatterning of graphene: First Concepts LOCAL ANODIC OXIATION STATIC PLOWING LITHOGRAPHY + AFM tip Water Gr substrate -Conductive substrate or at least contact at the graphene -conductive AFM tip -contact mode AFM -increased humidity -trench width ~ 30 nm -Contact mode -Lateral forces -Folding and crumbling AFM tip substrate A. J. M. Giesbers et al. , Solid State Communications 147, 366 (2008) S. Eilers et al. pss B 246, 2527 (2009)
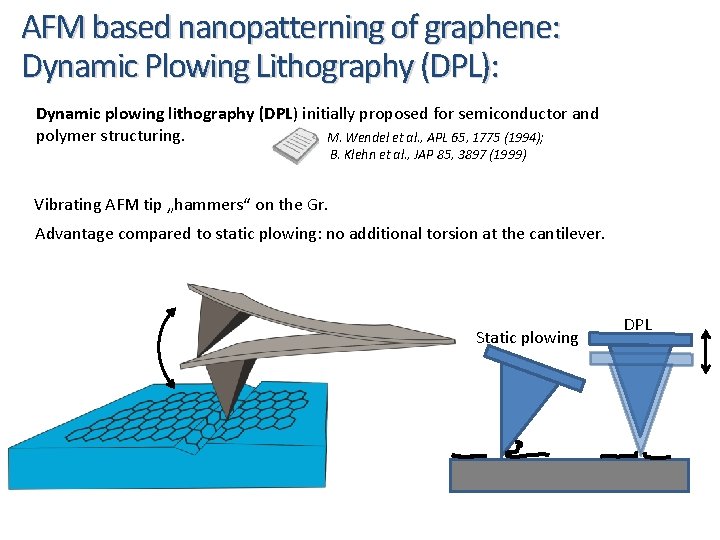
AFM based nanopatterning of graphene: Dynamic Plowing Lithography (DPL): Dynamic plowing lithography (DPL) initially proposed for semiconductor and polymer structuring. M. Wendel et al. , APL 65, 1775 (1994); B. Klehn et al. , JAP 85, 3897 (1999) Vibrating AFM tip „hammers“ on the Gr. Advantage compared to static plowing: no additional torsion at the cantilever. Static plowing DPL
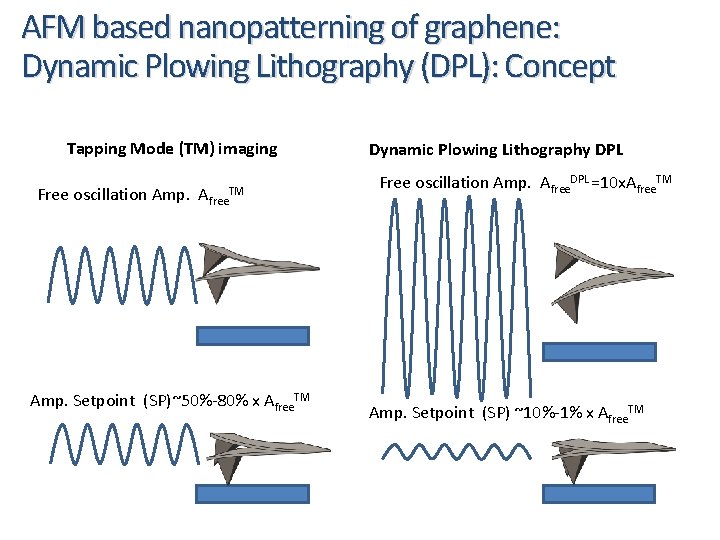
AFM based nanopatterning of graphene: Dynamic Plowing Lithography (DPL): Concept Tapping Mode (TM) imaging Free oscillation Amp. Afree. TM Amp. Setpoint (SP)~50%-80% x Afree. TM Dynamic Plowing Lithography DPL Free oscillation Amp. Afree. DPL =10 x. Afree. TM Amp. Setpoint (SP) ~10%-1% x Afree. TM
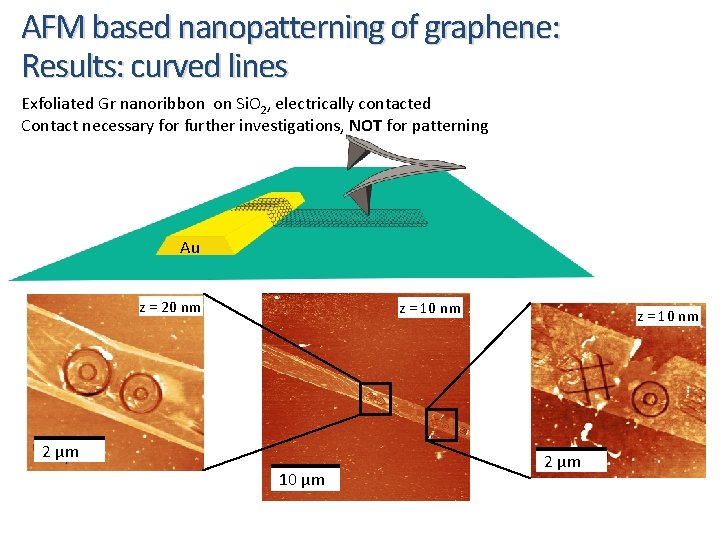
AFM based nanopatterning of graphene: Results: curved lines Exfoliated Gr nanoribbon on Si. O 2, electrically contacted Contact necessary for further investigations, NOT for patterning Au z = 20 nm z = 10 nm 2 µm 10 µm z = 10 nm 2 µm
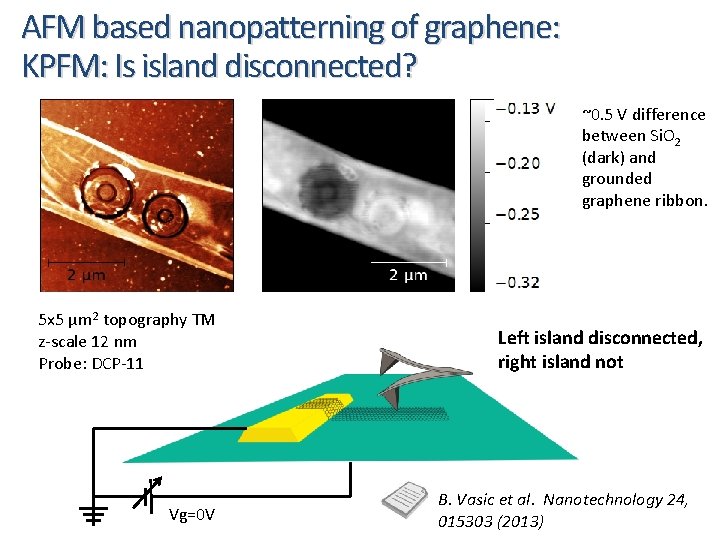
AFM based nanopatterning of graphene: KPFM: Is island disconnected? ~0. 5 V difference between Si. O 2 (dark) and grounded graphene ribbon. 5 x 5 µm 2 topography TM z-scale 12 nm Probe: DCP-11 Vg=0 V Left island disconnected, right island not B. Vasic et al. Nanotechnology 24, 015303 (2013)
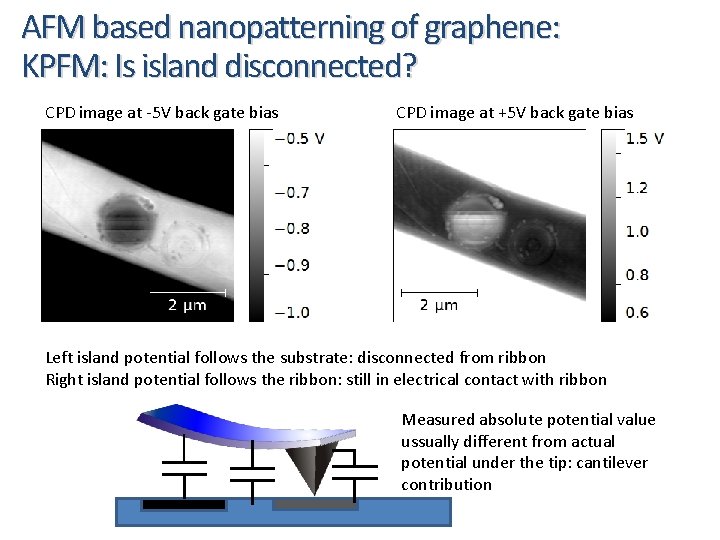
AFM based nanopatterning of graphene: KPFM: Is island disconnected? CPD image at -5 V back gate bias CPD image at +5 V back gate bias Left island potential follows the substrate: disconnected from ribbon Right island potential follows the ribbon: still in electrical contact with ribbon Measured absolute potential value ussually different from actual potential under the tip: cantilever contribution
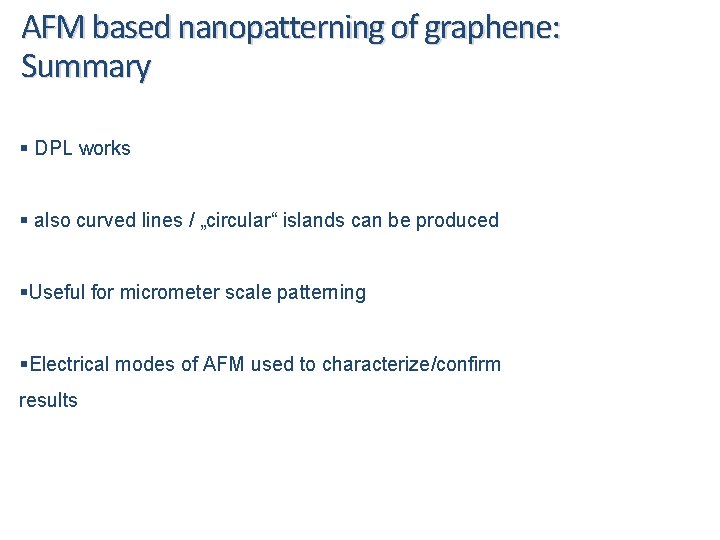
AFM based nanopatterning of graphene: Summary § DPL works § also curved lines / „circular“ islands can be produced §Useful for micrometer scale patterning §Electrical modes of AFM used to characterize/confirm results
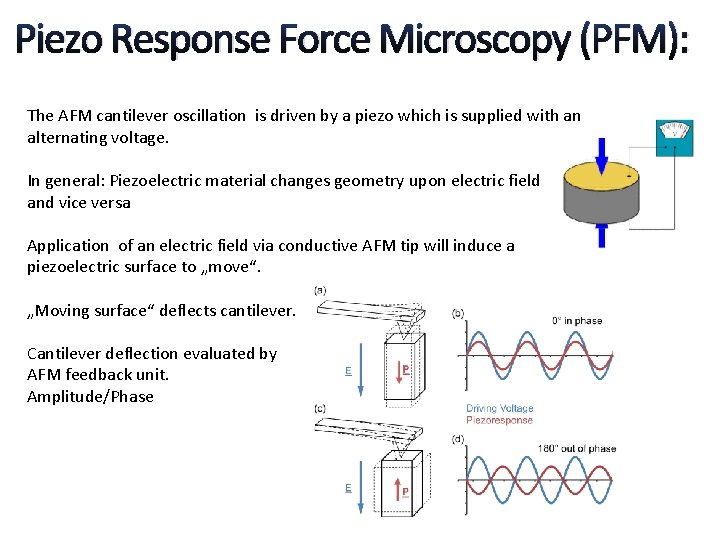
Piezo Response Force Microscopy (PFM): The AFM cantilever oscillation is driven by a piezo which is supplied with an alternating voltage. In general: Piezoelectric material changes geometry upon electric field and vice versa Application of an electric field via conductive AFM tip will induce a piezoelectric surface to „move“. „Moving surface“ deflects cantilever. Cantilever deflection evaluated by AFM feedback unit. Amplitude/Phase
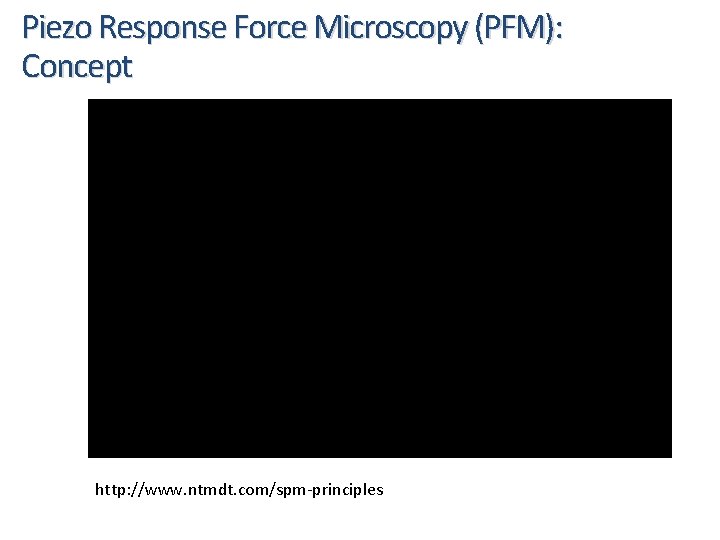
Piezo Response Force Microscopy (PFM): Concept http: //www. ntmdt. com/spm-principles
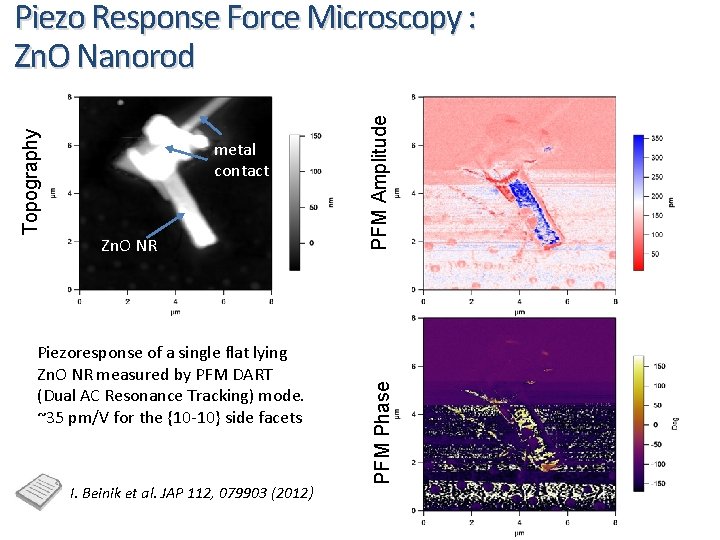
Zn. O NR Piezoresponse of a single flat lying Zn. O NR measured by PFM DART (Dual AC Resonance Tracking) mode. ~35 pm/V for the {10 -10} side facets I. Beinik et al. JAP 112, 079903 (2012) PFM Amplitude metal contact PFM Phase Topography Piezo Response Force Microscopy : Zn. O Nanorod
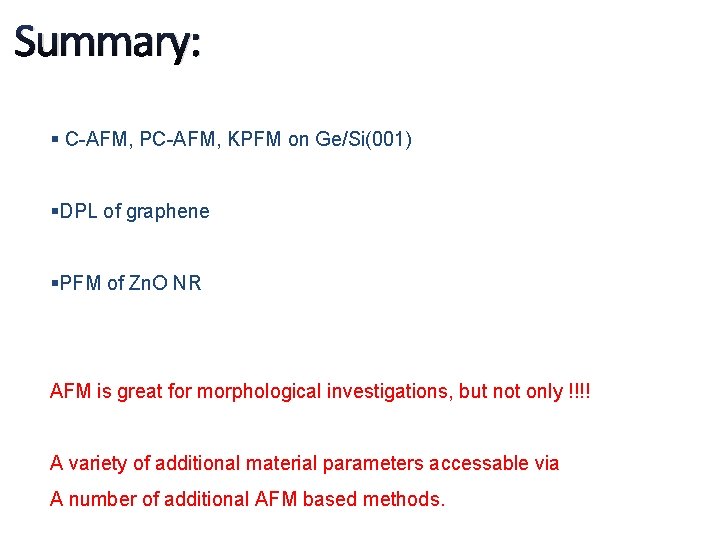
Summary: § C-AFM, PC-AFM, KPFM on Ge/Si(001) §DPL of graphene §PFM of Zn. O NR AFM is great for morphological investigations, but not only !!!! A variety of additional material parameters accessable via A number of additional AFM based methods.
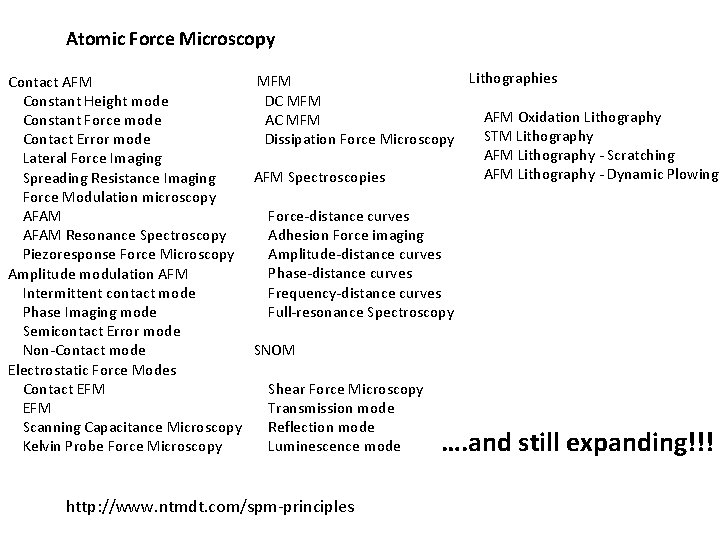
Atomic Force Microscopy Contact AFM Constant Height mode Constant Force mode Contact Error mode Lateral Force Imaging Spreading Resistance Imaging Force Modulation microscopy AFAM Resonance Spectroscopy Piezoresponse Force Microscopy Amplitude modulation AFM Intermittent contact mode Phase Imaging mode Semicontact Error mode Non-Contact mode Electrostatic Force Modes Contact EFM Scanning Capacitance Microscopy Kelvin Probe Force Microscopy Lithographies MFM DC MFM AFM Oxidation Lithography AC MFM Dissipation Force Microscopy STM Lithography AFM Lithography - Scratching AFM Lithography - Dynamic Plowing AFM Spectroscopies Force-distance curves Adhesion Force imaging Amplitude-distance curves Phase-distance curves Frequency-distance curves Full-resonance Spectroscopy SNOM Shear Force Microscopy Transmission mode Reflection mode Luminescence mode http: //www. ntmdt. com/spm-principles …. and still expanding!!!
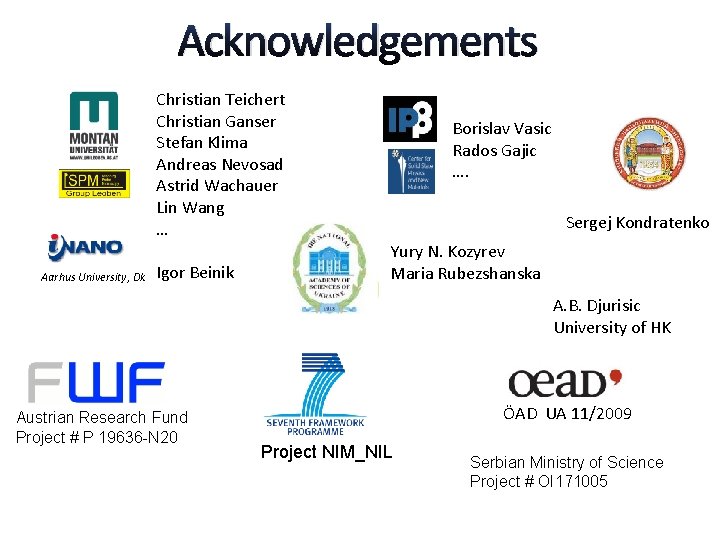
Acknowledgements Christian Teichert Christian Ganser Stefan Klima Andreas Nevosad Astrid Wachauer Lin Wang … Aarhus University, Dk Igor Beinik Borislav Vasic Rados Gajic …. Sergej Kondratenko Yury N. Kozyrev Maria Rubezshanska A. B. Djurisic University of HK Austrian Research Fund Project # P 19636 -N 20 ÖAD UA 11/2009 Project NIM_NIL Serbian Ministry of Science Project # OI 171005
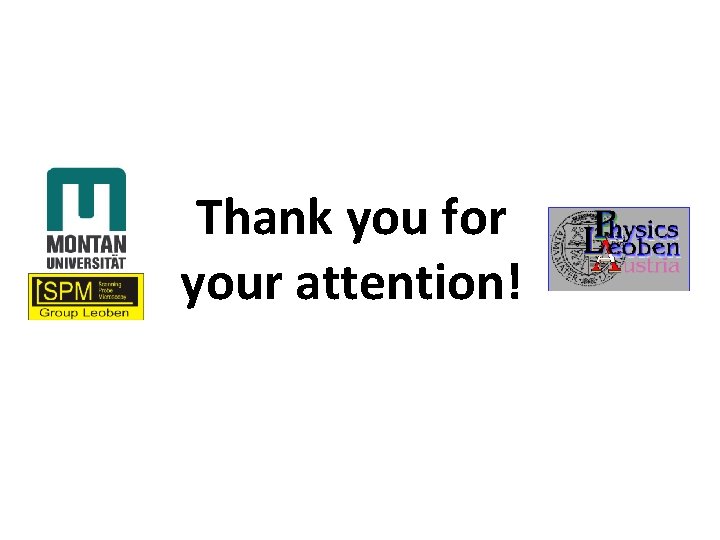
Thank you for your attention!
Atomic force microscope
Versatile storage systems
Dvb.org
Versatile mathematical concept
Leonardo da vinci personality
Versatile records
Relative formula mass of hcl
Trends of periodic table
Ionic radius examples
Is atomic mass and relative atomic mass the same
Whats the difference between atomic mass and atomic number
Atomic number vs atomic radius
Light microscope vs electron microscope
Microscope mania compound light microscope
Tool makers microscope diagram
Metrology and measurements subject code
Potter's tool is data cleaning tool
Hình ảnh bộ gõ cơ thể búng tay
Lp html
Bổ thể
Tỉ lệ cơ thể trẻ em
Voi kéo gỗ như thế nào
Tư thế worms-breton
Alleluia hat len nguoi oi
Các môn thể thao bắt đầu bằng tiếng đua
Thế nào là hệ số cao nhất
Các châu lục và đại dương trên thế giới
Công thức tính thế năng
Trời xanh đây là của chúng ta thể thơ
Cách giải mật thư tọa độ