Jet Engine Materials A quick overview of the
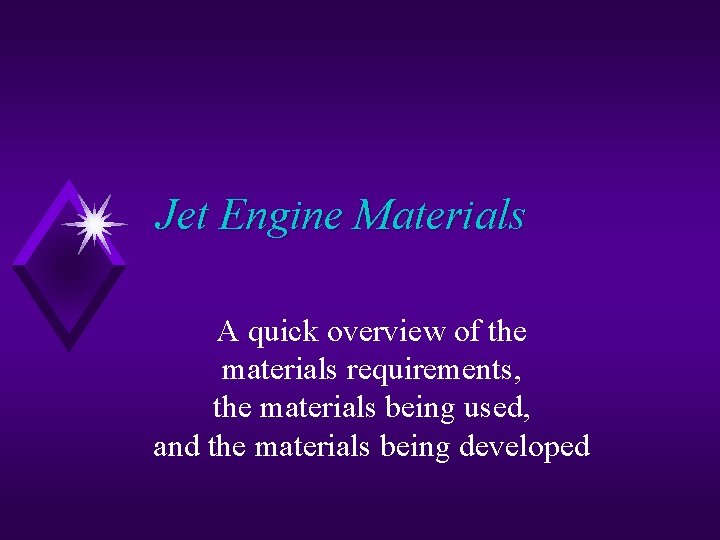
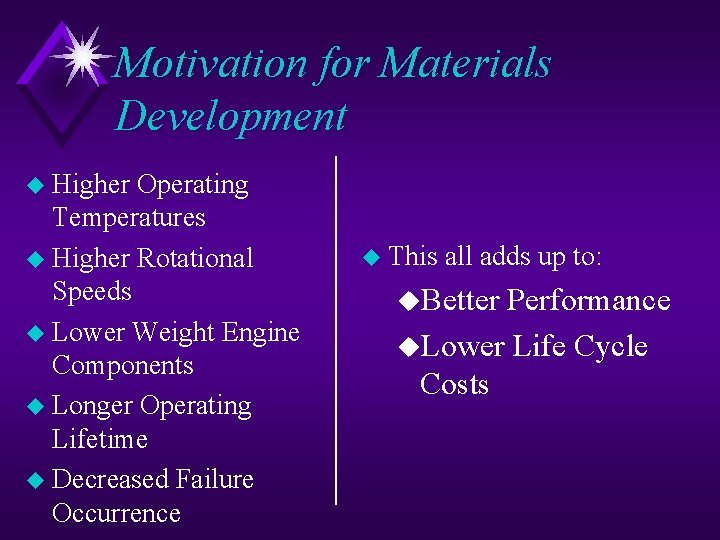
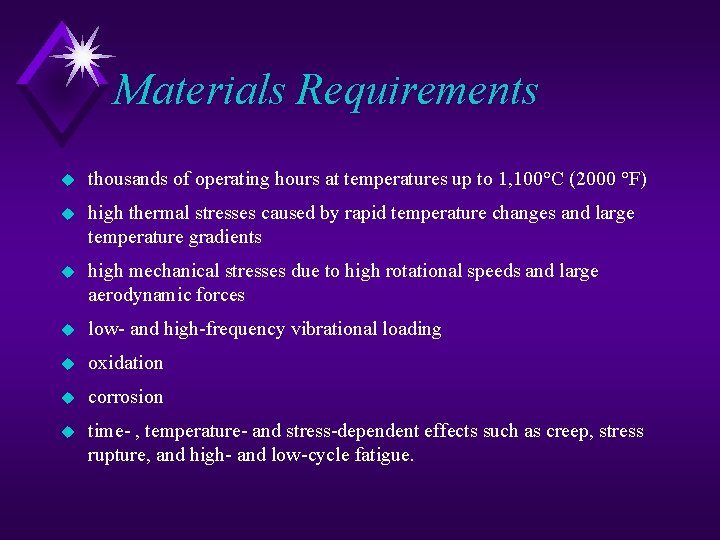
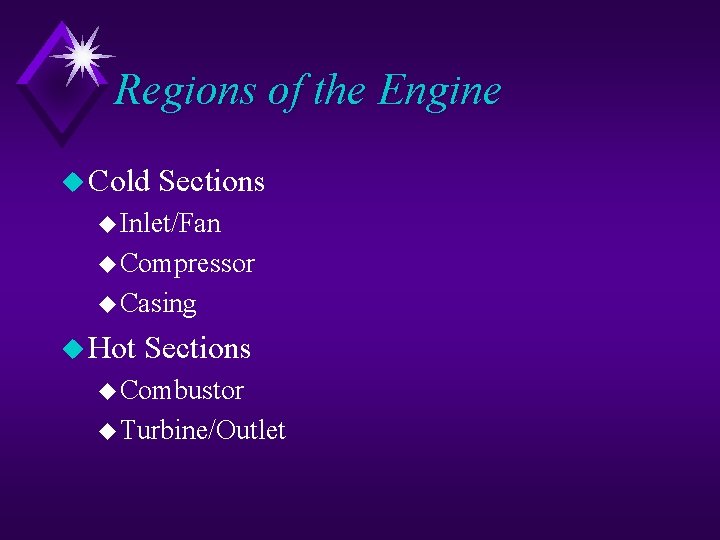
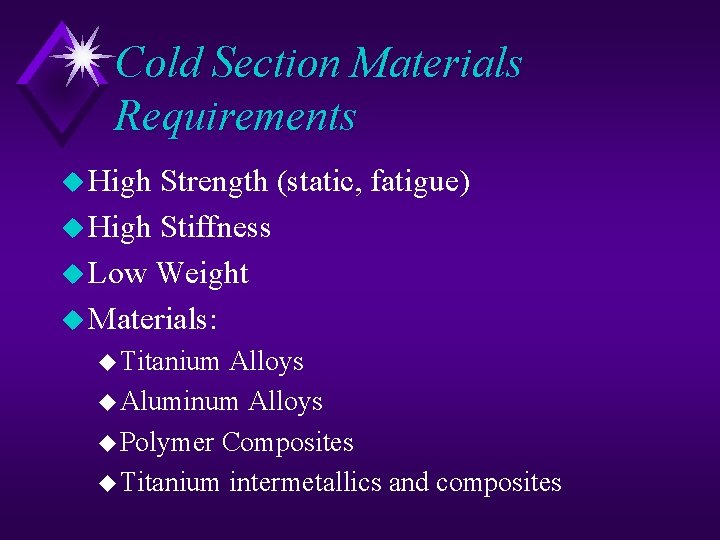
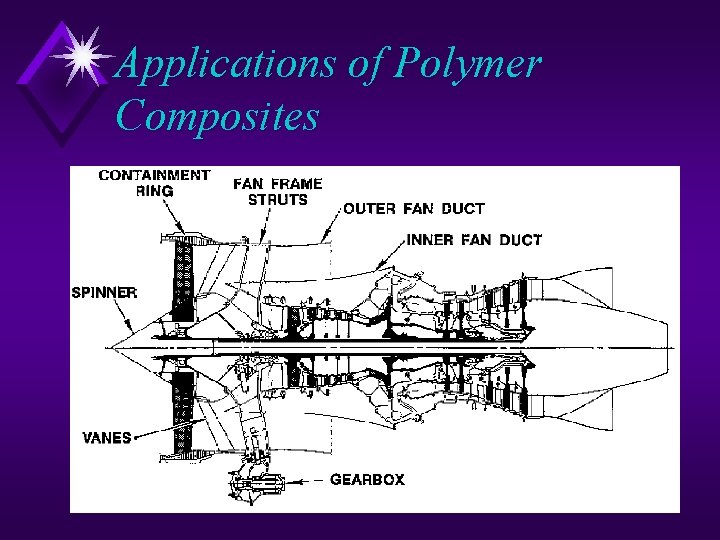
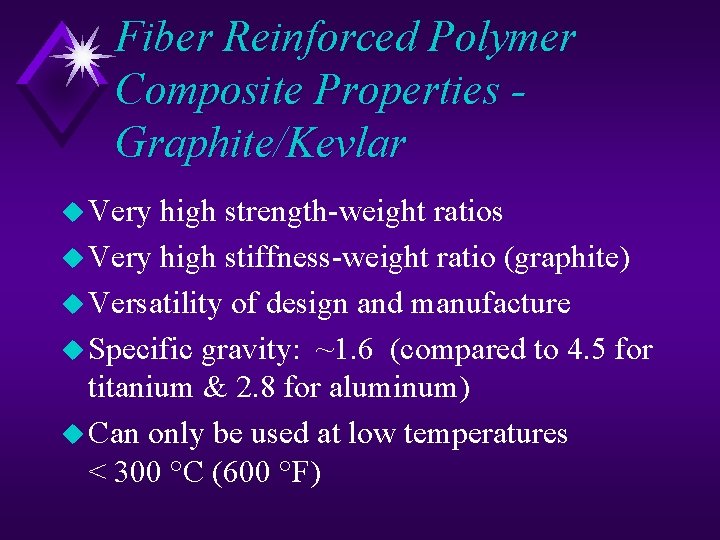
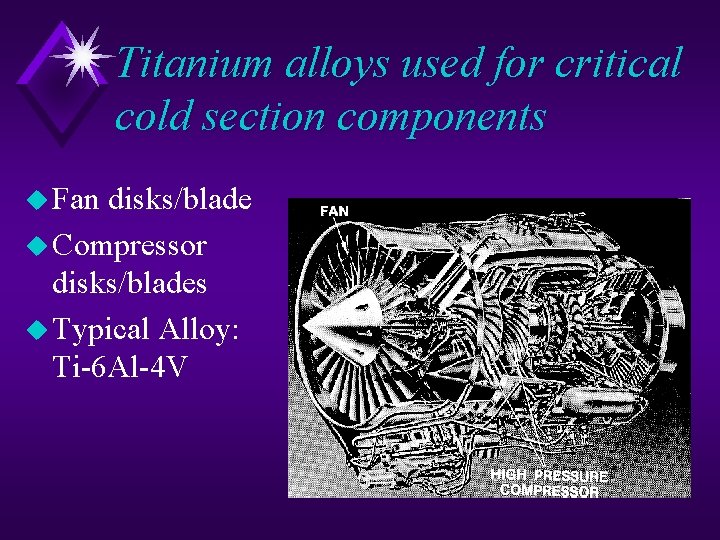
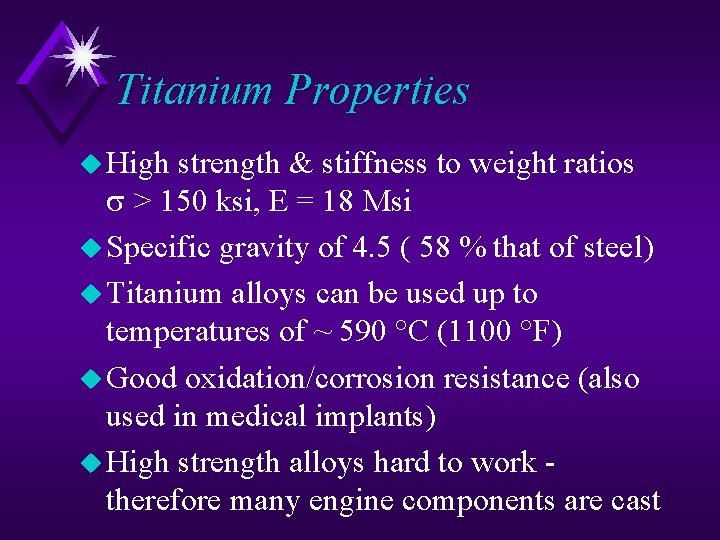
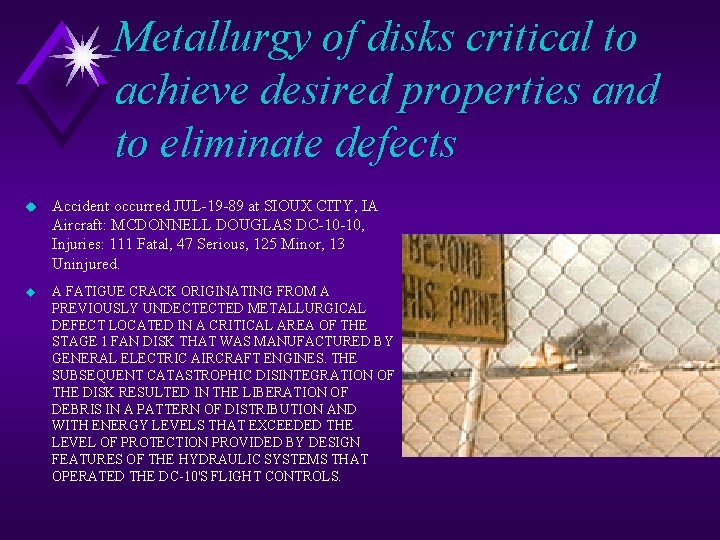
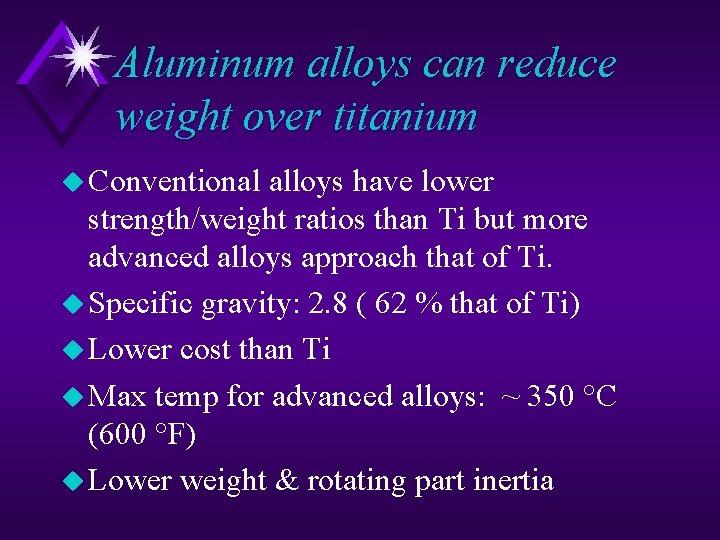
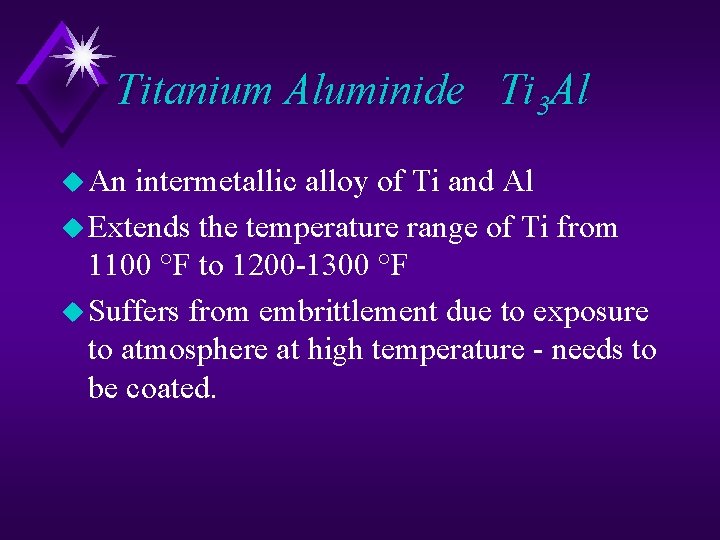
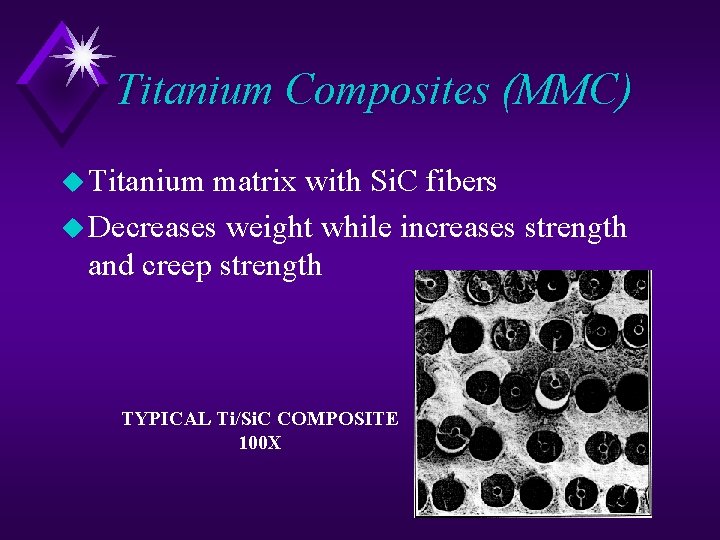
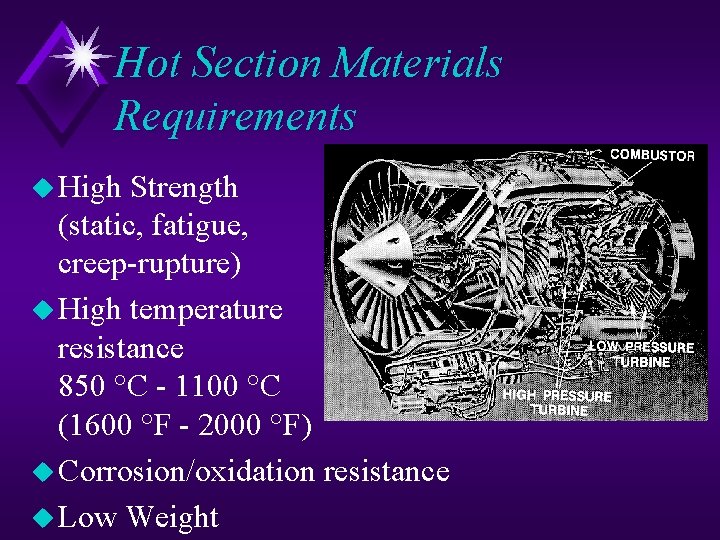
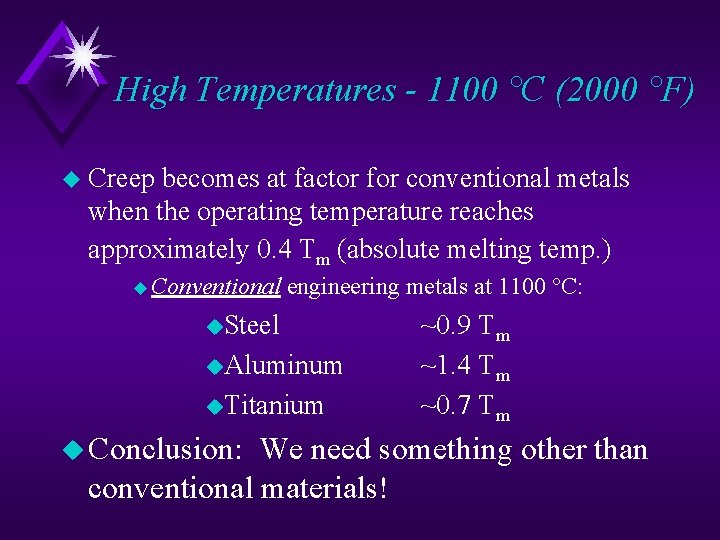
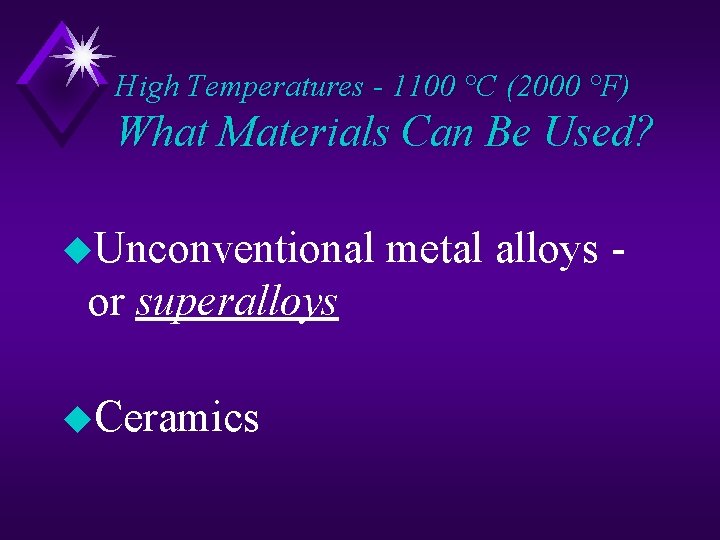
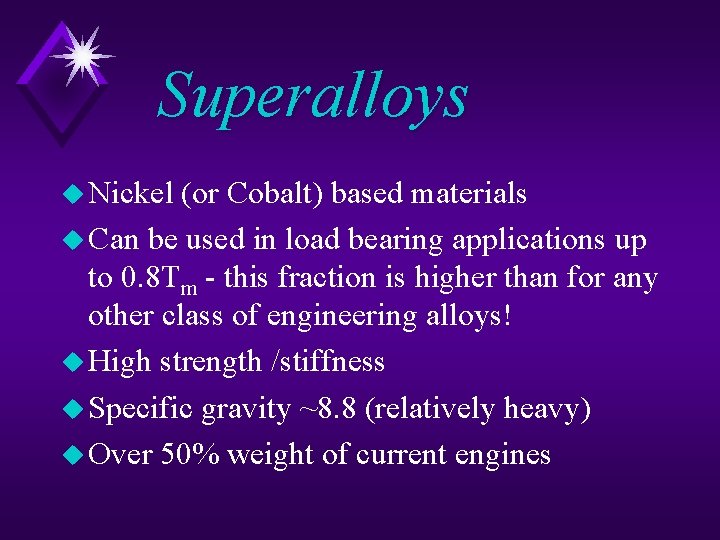
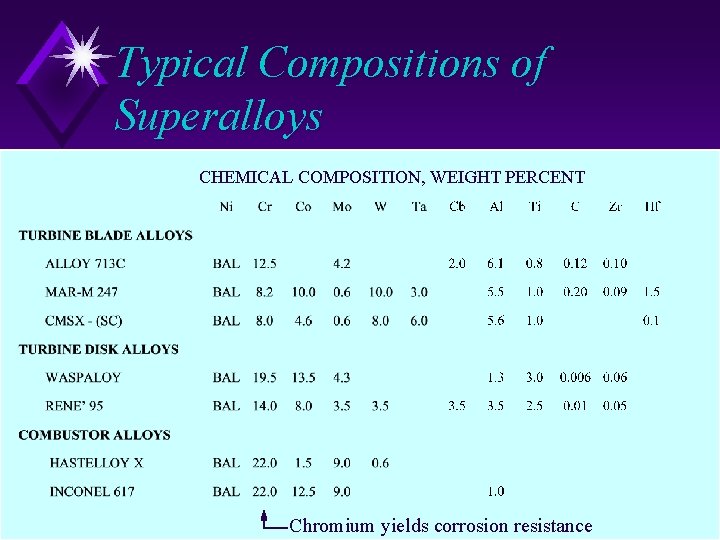
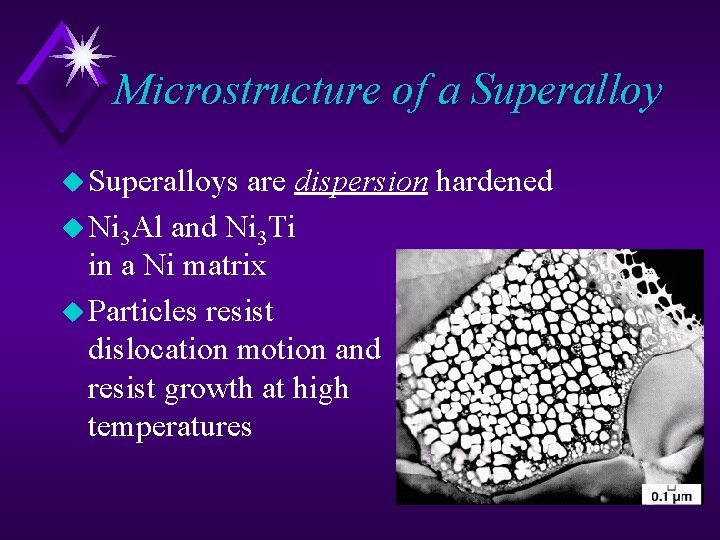
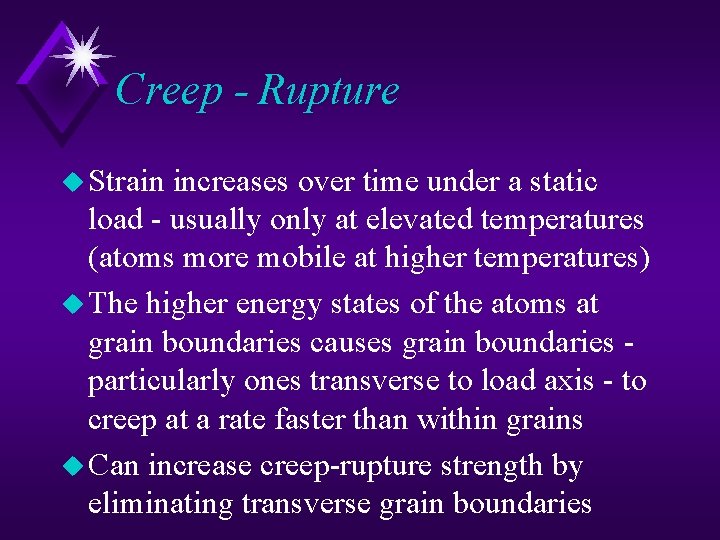
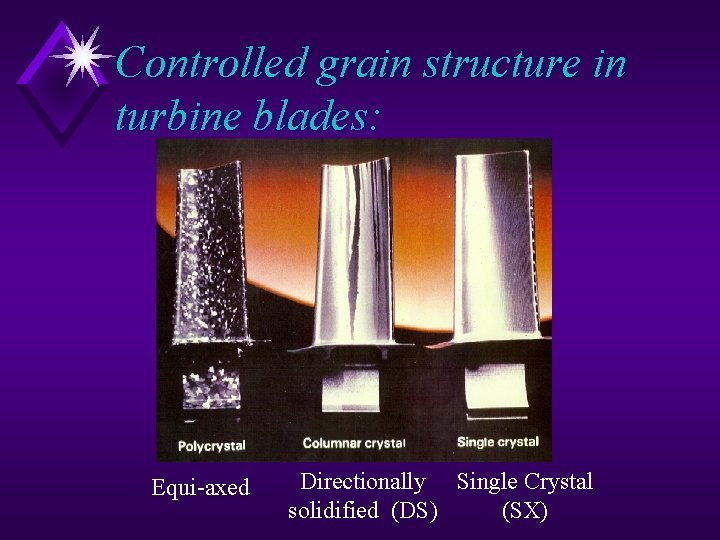
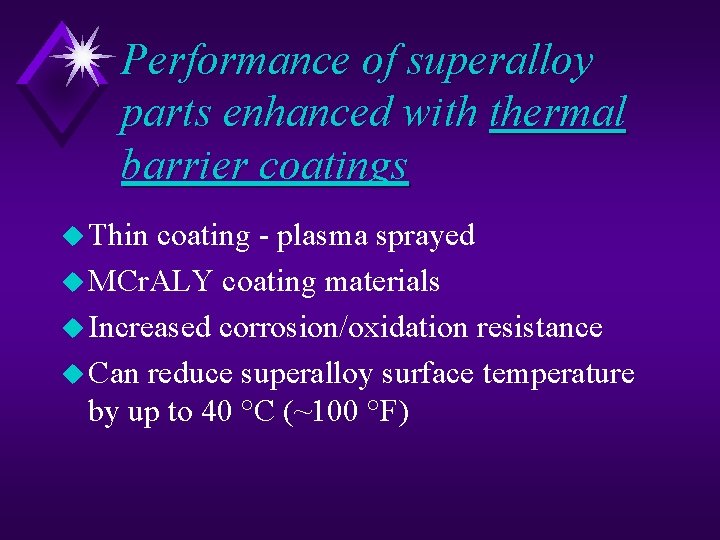
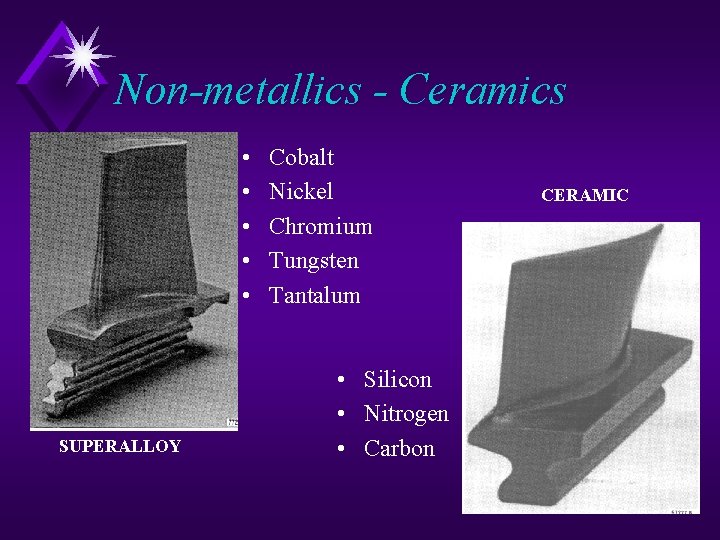
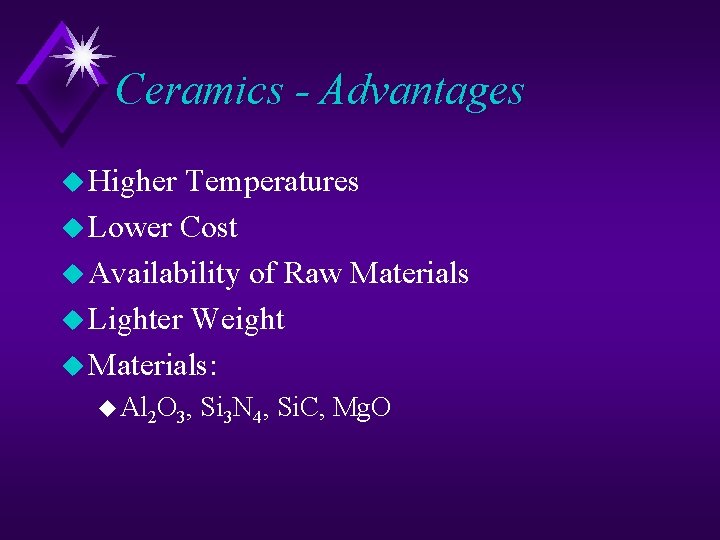
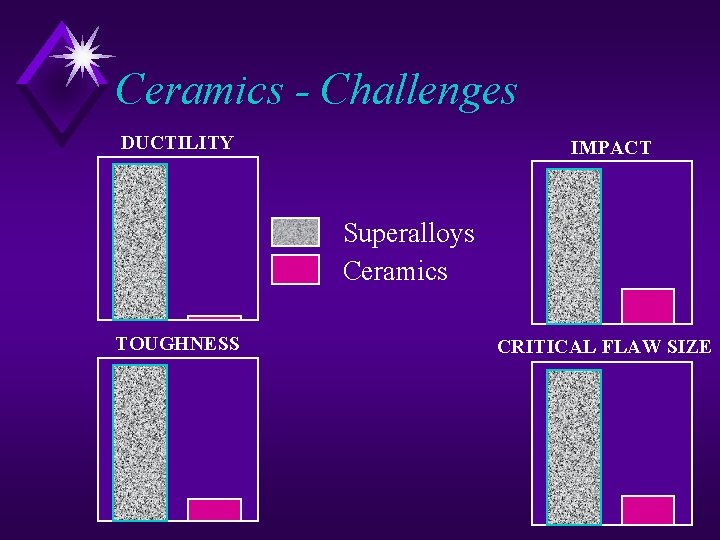
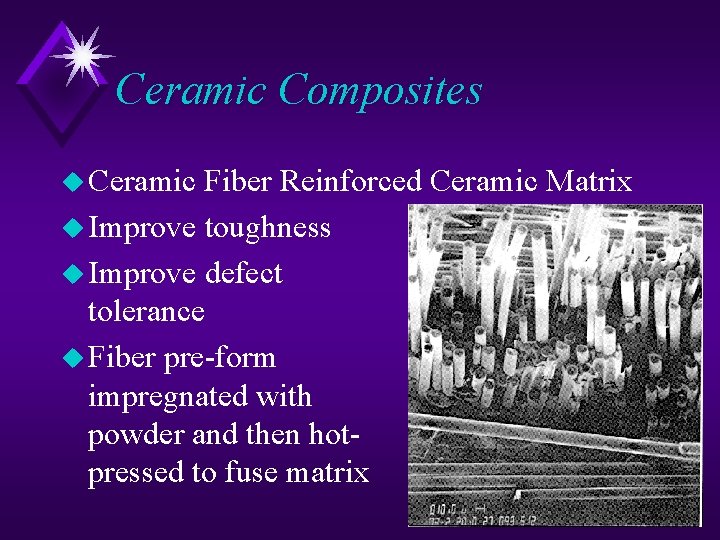
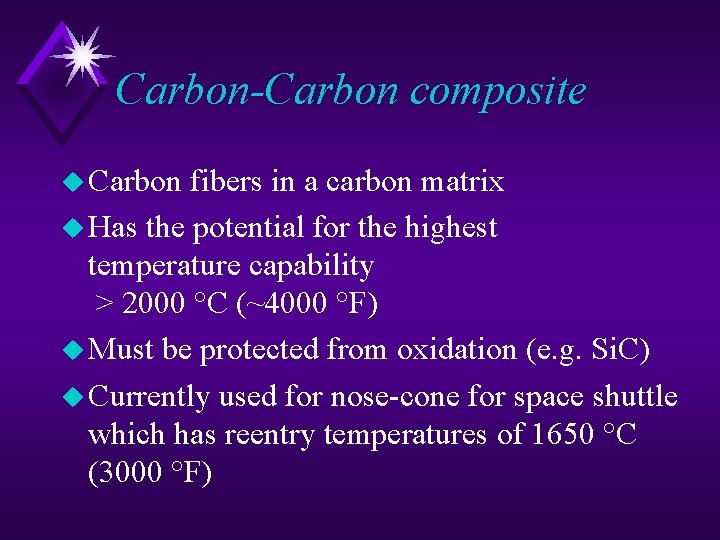
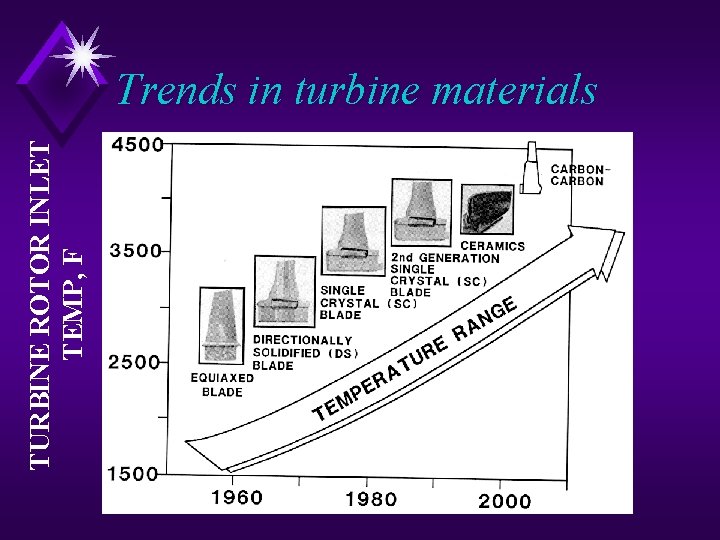
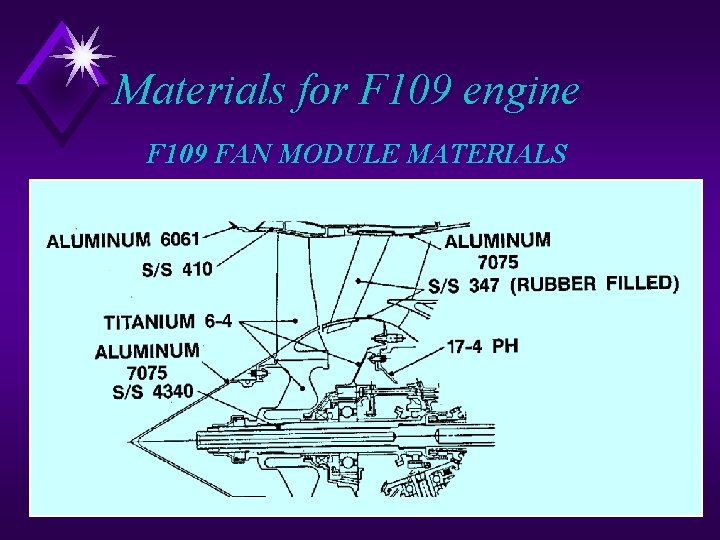
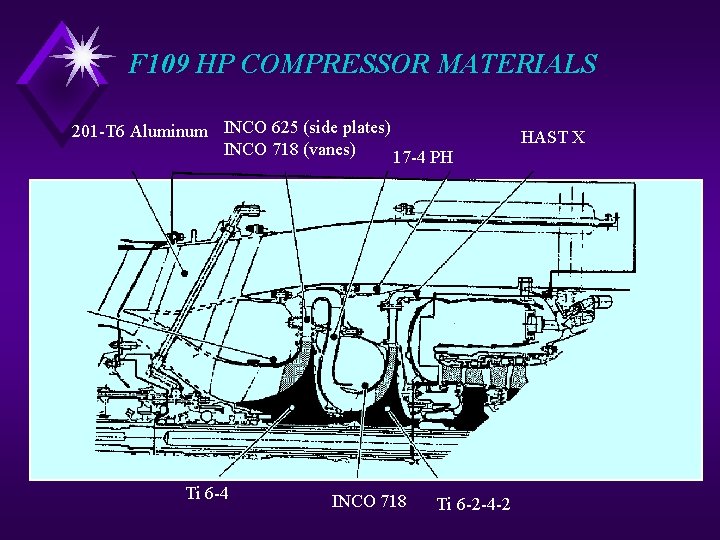
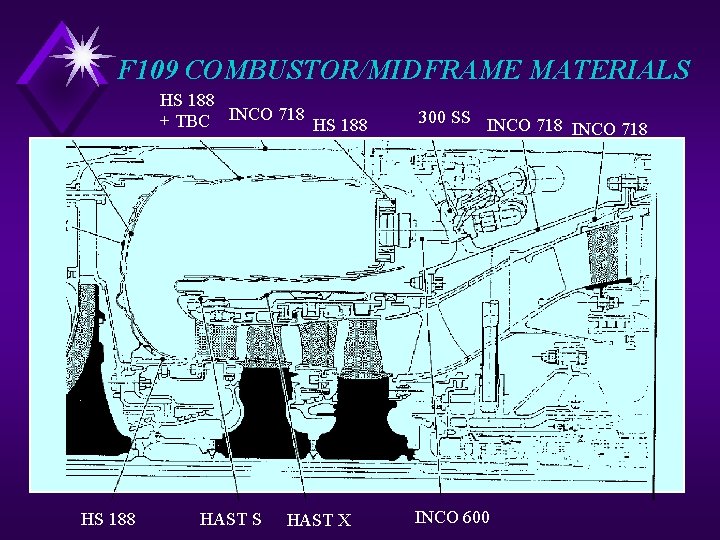
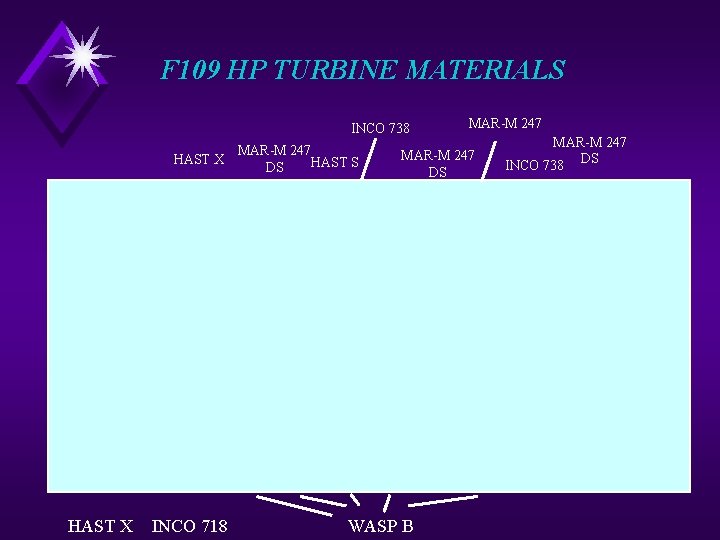
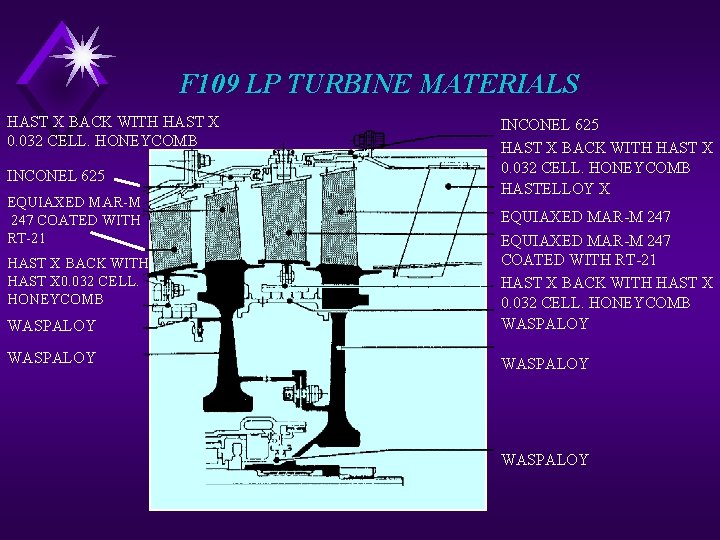
- Slides: 33
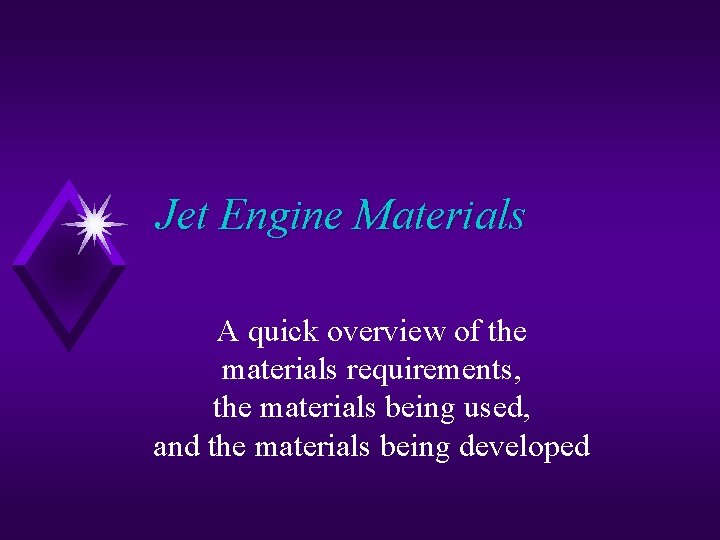
Jet Engine Materials A quick overview of the materials requirements, the materials being used, and the materials being developed
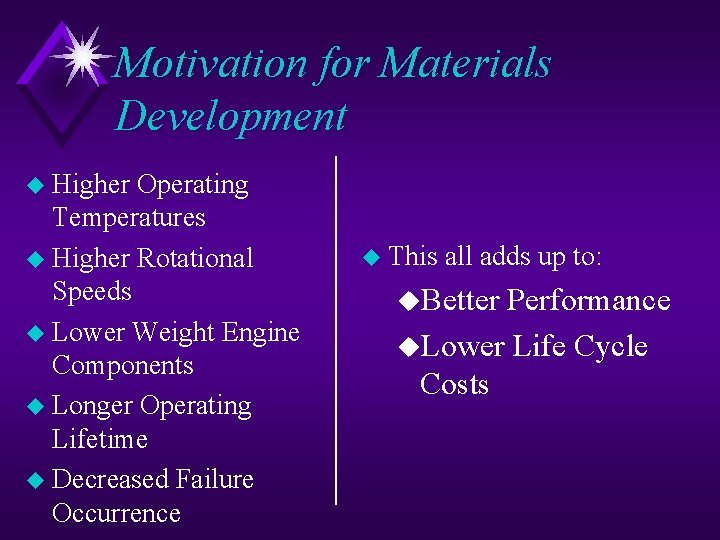
Motivation for Materials Development u Higher Operating Temperatures u Higher Rotational Speeds u Lower Weight Engine Components u Longer Operating Lifetime u Decreased Failure Occurrence u This all adds up to: u. Better Performance u. Lower Life Cycle Costs
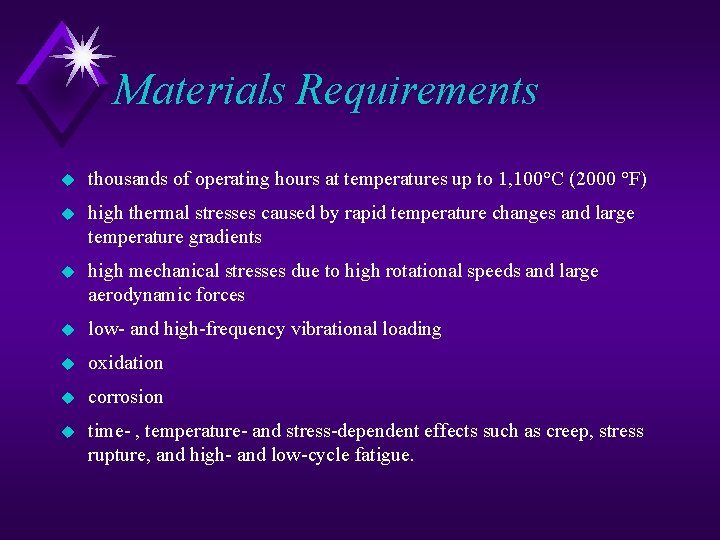
Materials Requirements u thousands of operating hours at temperatures up to 1, 100°C (2000 °F) u high thermal stresses caused by rapid temperature changes and large temperature gradients u high mechanical stresses due to high rotational speeds and large aerodynamic forces u low- and high-frequency vibrational loading u oxidation u corrosion u time- , temperature- and stress-dependent effects such as creep, stress rupture, and high- and low-cycle fatigue.
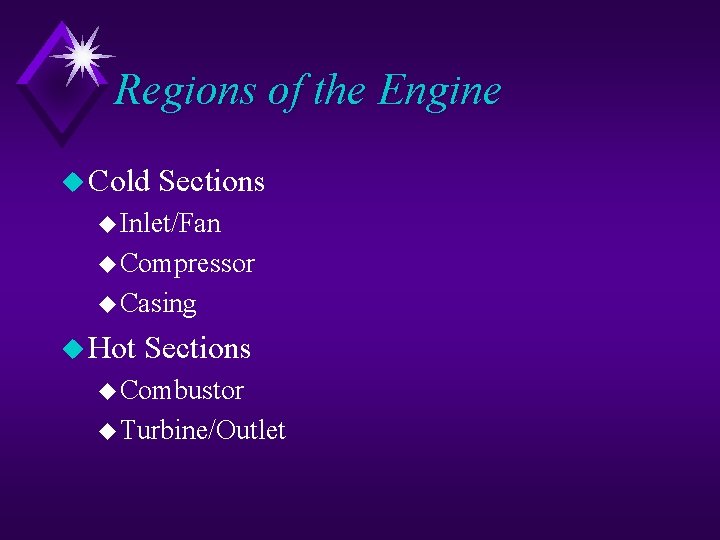
Regions of the Engine u Cold Sections u Inlet/Fan u Compressor u Casing u Hot Sections u Combustor u Turbine/Outlet
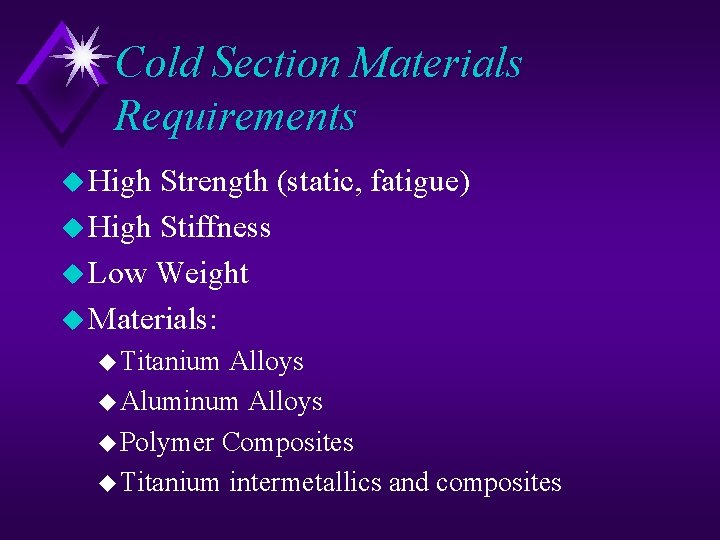
Cold Section Materials Requirements u High Strength (static, fatigue) u High Stiffness u Low Weight u Materials: u Titanium Alloys u Aluminum Alloys u Polymer Composites u Titanium intermetallics and composites
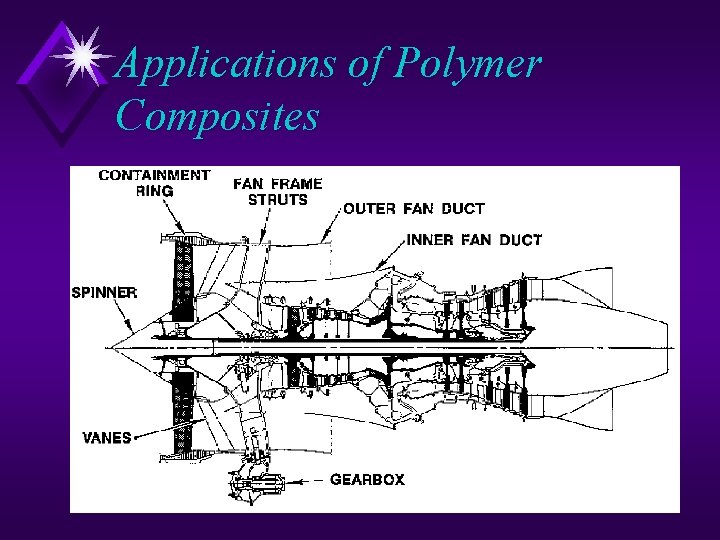
Applications of Polymer Composites
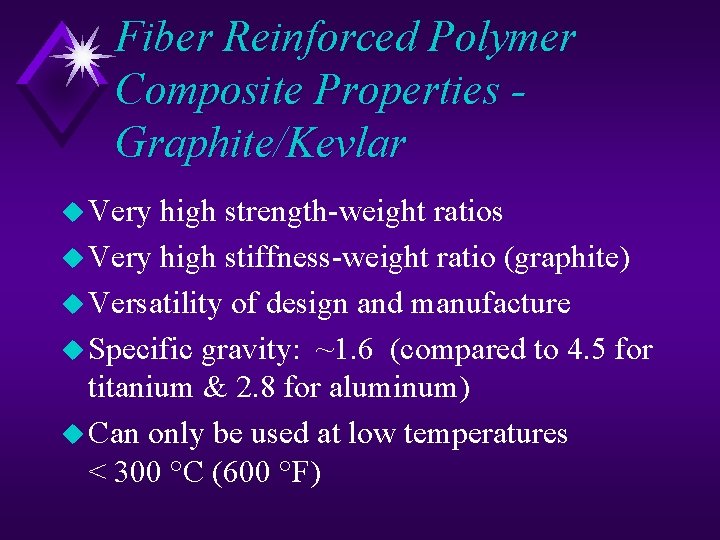
Fiber Reinforced Polymer Composite Properties Graphite/Kevlar u Very high strength-weight ratios u Very high stiffness-weight ratio (graphite) u Versatility of design and manufacture u Specific gravity: ~1. 6 (compared to 4. 5 for titanium & 2. 8 for aluminum) u Can only be used at low temperatures < 300 °C (600 °F)
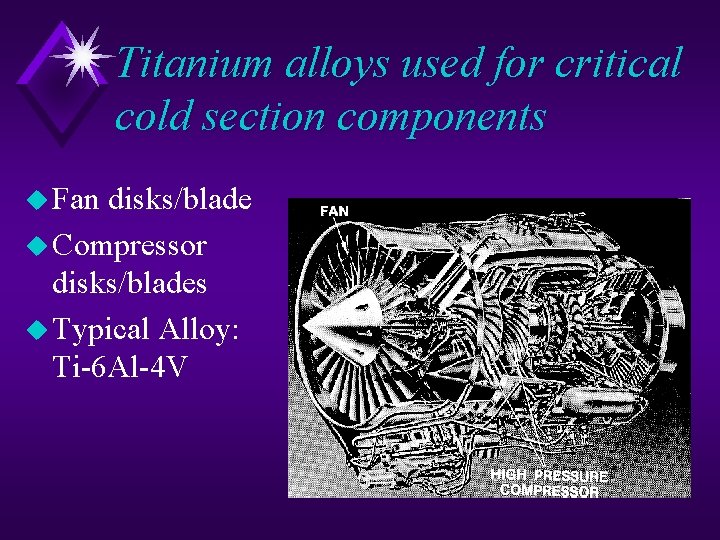
Titanium alloys used for critical cold section components u Fan disks/blade u Compressor disks/blades u Typical Alloy: Ti-6 Al-4 V
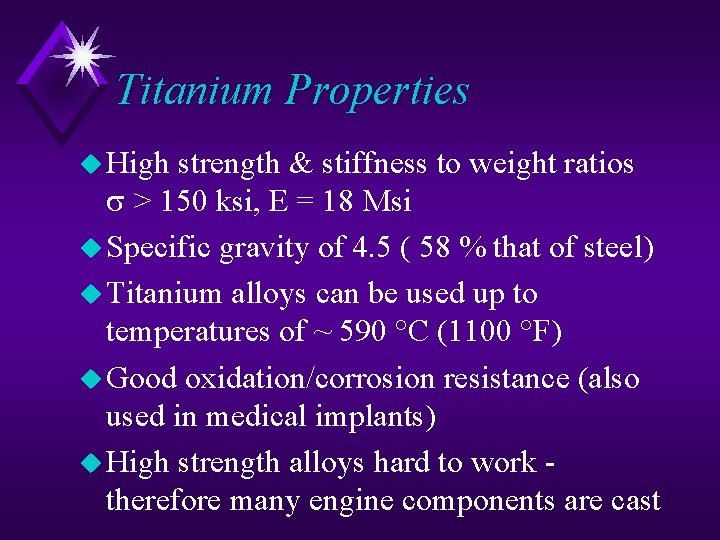
Titanium Properties u High strength & stiffness to weight ratios > 150 ksi, E = 18 Msi u Specific gravity of 4. 5 ( 58 % that of steel) u Titanium alloys can be used up to temperatures of ~ 590 °C (1100 °F) u Good oxidation/corrosion resistance (also used in medical implants) u High strength alloys hard to work therefore many engine components are cast
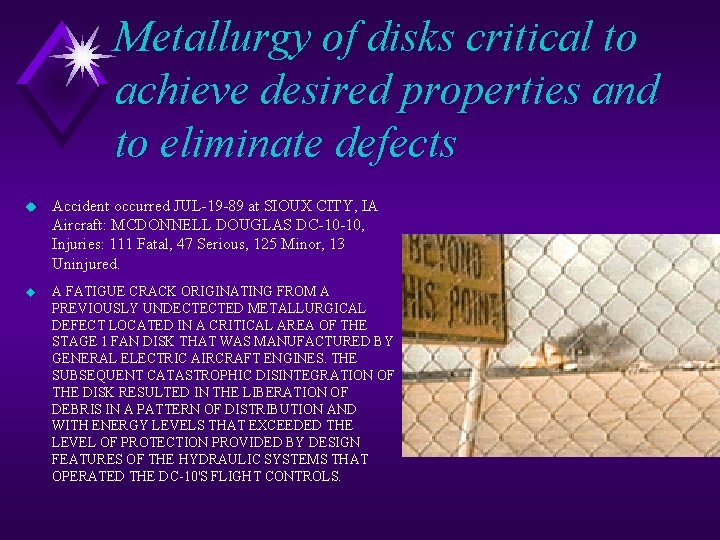
Metallurgy of disks critical to achieve desired properties and to eliminate defects u Accident occurred JUL-19 -89 at SIOUX CITY, IA Aircraft: MCDONNELL DOUGLAS DC-10 -10, Injuries: 111 Fatal, 47 Serious, 125 Minor, 13 Uninjured. u A FATIGUE CRACK ORIGINATING FROM A PREVIOUSLY UNDECTECTED METALLURGICAL DEFECT LOCATED IN A CRITICAL AREA OF THE STAGE 1 FAN DISK THAT WAS MANUFACTURED BY GENERAL ELECTRIC AIRCRAFT ENGINES. THE SUBSEQUENT CATASTROPHIC DISINTEGRATION OF THE DISK RESULTED IN THE LIBERATION OF DEBRIS IN A PATTERN OF DISTRIBUTION AND WITH ENERGY LEVELS THAT EXCEEDED THE LEVEL OF PROTECTION PROVIDED BY DESIGN FEATURES OF THE HYDRAULIC SYSTEMS THAT OPERATED THE DC-10'S FLIGHT CONTROLS.
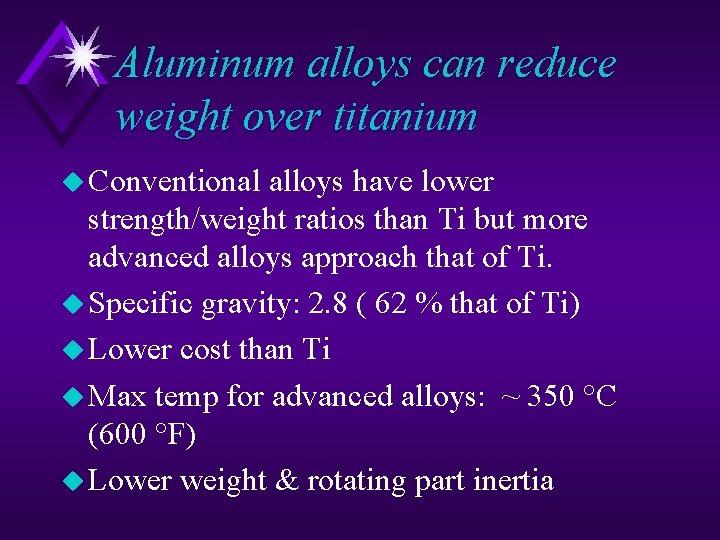
Aluminum alloys can reduce weight over titanium u Conventional alloys have lower strength/weight ratios than Ti but more advanced alloys approach that of Ti. u Specific gravity: 2. 8 ( 62 % that of Ti) u Lower cost than Ti u Max temp for advanced alloys: ~ 350 °C (600 °F) u Lower weight & rotating part inertia
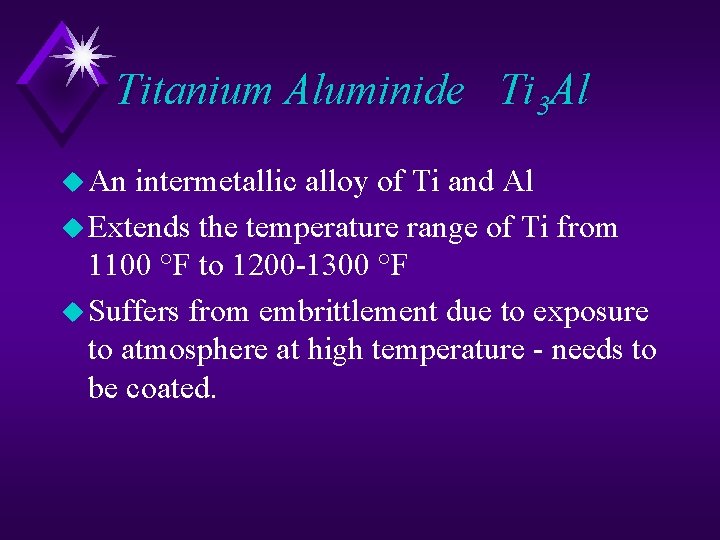
Titanium Aluminide Ti 3 Al u An intermetallic alloy of Ti and Al u Extends the temperature range of Ti from 1100 °F to 1200 -1300 °F u Suffers from embrittlement due to exposure to atmosphere at high temperature - needs to be coated.
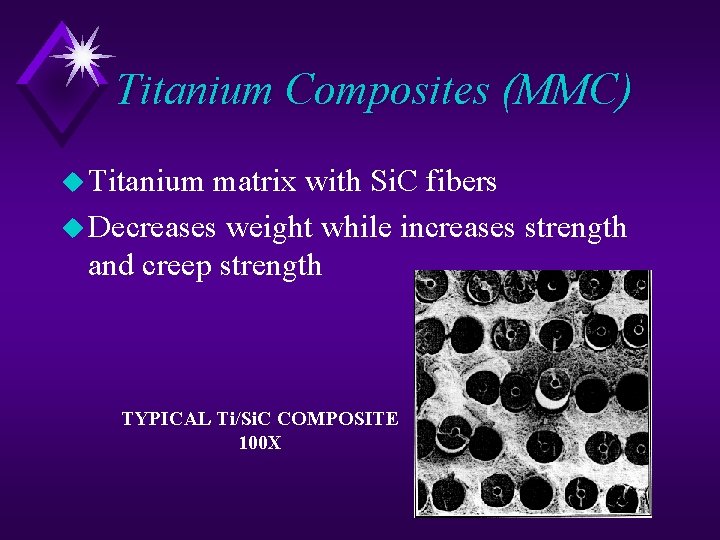
Titanium Composites (MMC) u Titanium matrix with Si. C fibers u Decreases weight while increases strength and creep strength TYPICAL Ti/Si. C COMPOSITE 100 X
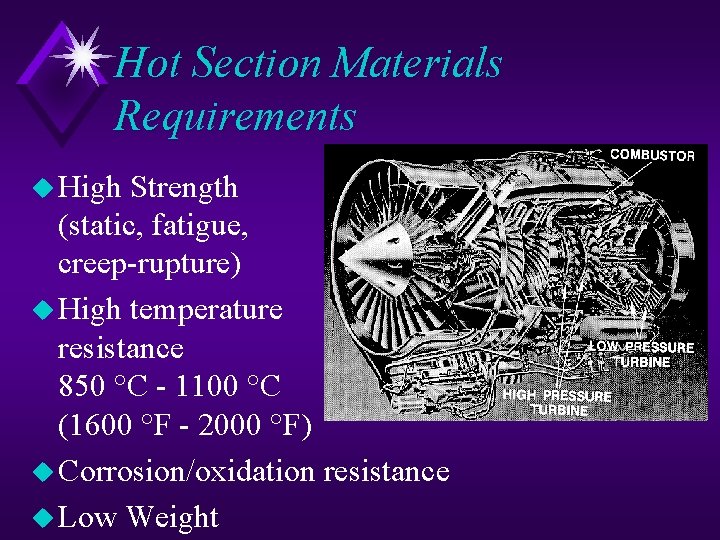
Hot Section Materials Requirements u High Strength (static, fatigue, creep-rupture) u High temperature resistance 850 °C - 1100 °C (1600 °F - 2000 °F) u Corrosion/oxidation resistance u Low Weight
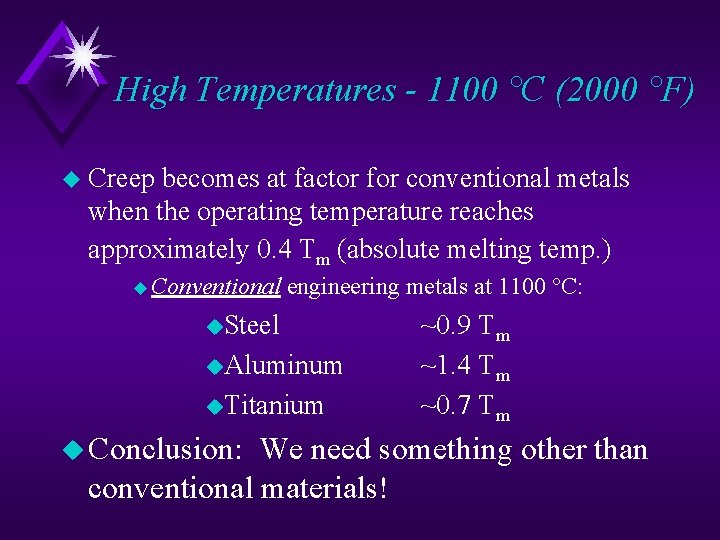
High Temperatures - 1100 °C (2000 °F) u Creep becomes at factor for conventional metals when the operating temperature reaches approximately 0. 4 Tm (absolute melting temp. ) u Conventional engineering metals at 1100 °C: u. Steel u. Aluminum u. Titanium u Conclusion: ~0. 9 Tm ~1. 4 Tm ~0. 7 Tm We need something other than conventional materials!
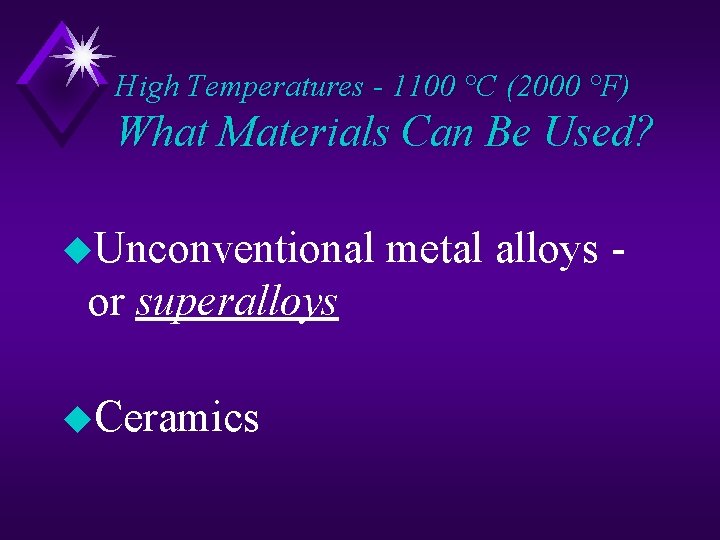
High Temperatures - 1100 °C (2000 °F) What Materials Can Be Used? u. Unconventional or superalloys u. Ceramics metal alloys -
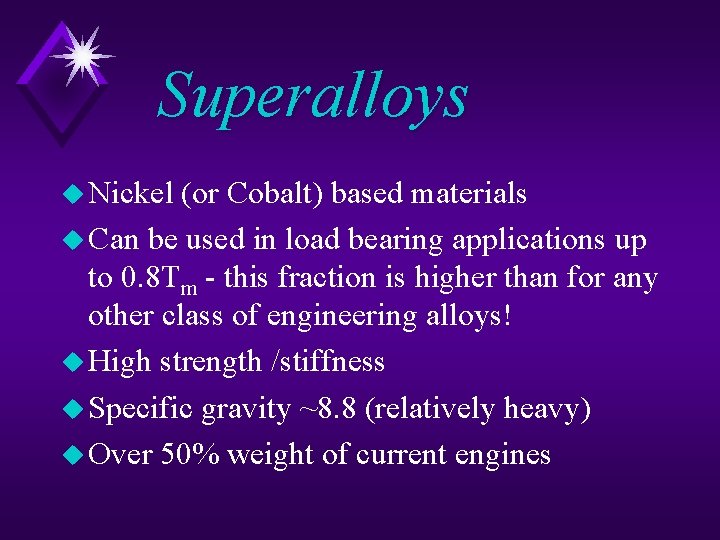
Superalloys u Nickel (or Cobalt) based materials u Can be used in load bearing applications up to 0. 8 Tm - this fraction is higher than for any other class of engineering alloys! u High strength /stiffness u Specific gravity ~8. 8 (relatively heavy) u Over 50% weight of current engines
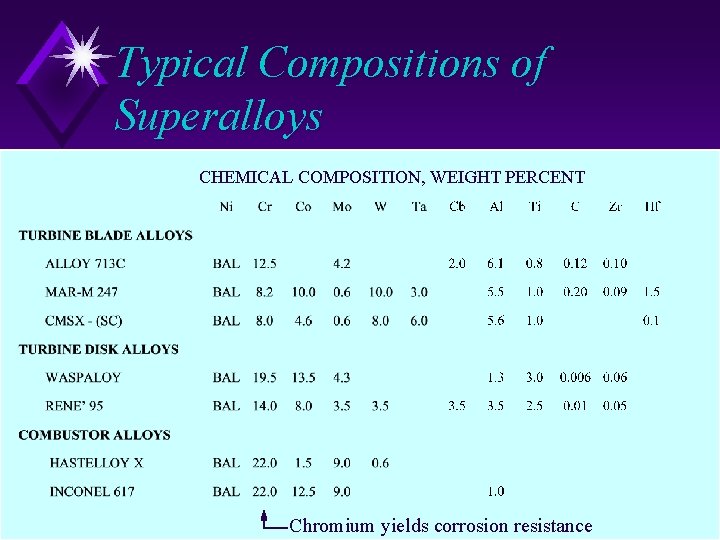
Typical Compositions of Superalloys CHEMICAL COMPOSITION, WEIGHT PERCENT Chromium yields corrosion resistance
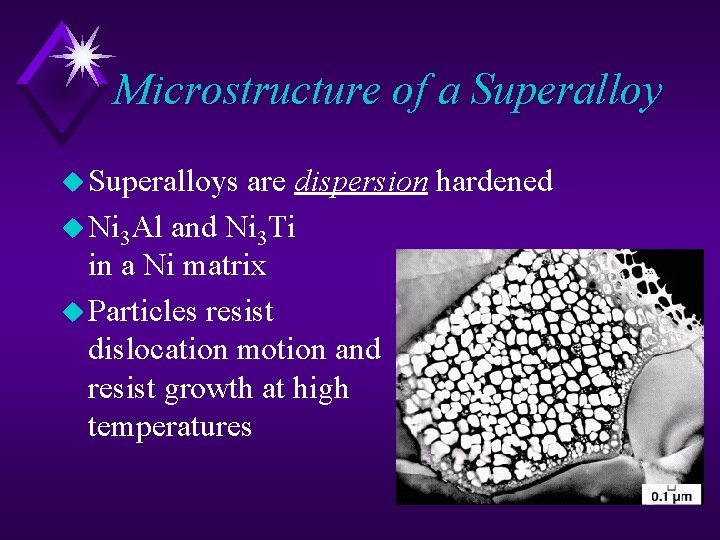
Microstructure of a Superalloy u Superalloys are dispersion hardened u Ni 3 Al and Ni 3 Ti in a Ni matrix u Particles resist dislocation motion and resist growth at high temperatures
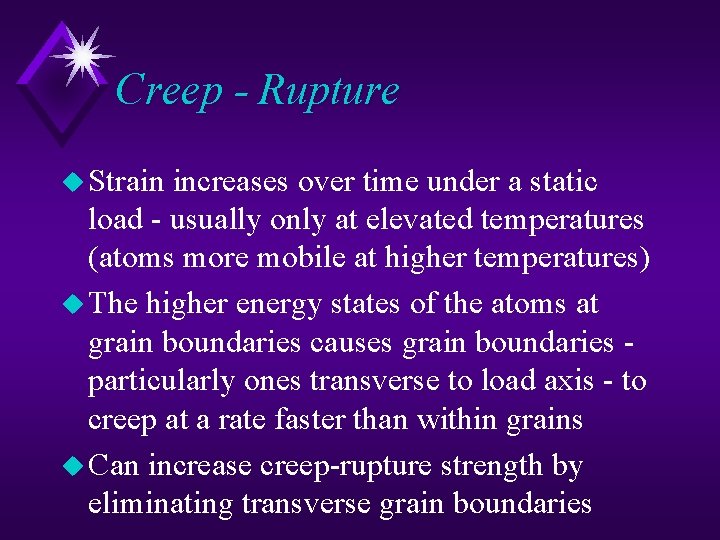
Creep - Rupture u Strain increases over time under a static load - usually only at elevated temperatures (atoms more mobile at higher temperatures) u The higher energy states of the atoms at grain boundaries causes grain boundaries particularly ones transverse to load axis - to creep at a rate faster than within grains u Can increase creep-rupture strength by eliminating transverse grain boundaries
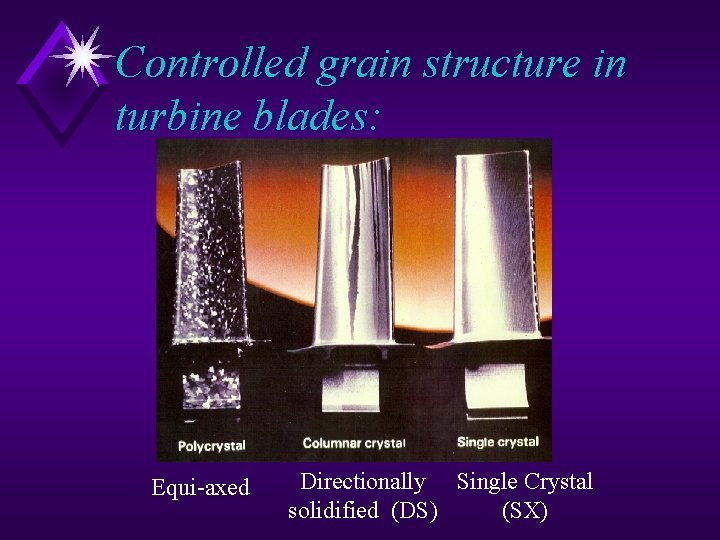
Controlled grain structure in turbine blades: Equi-axed Directionally Single Crystal solidified (DS) (SX)
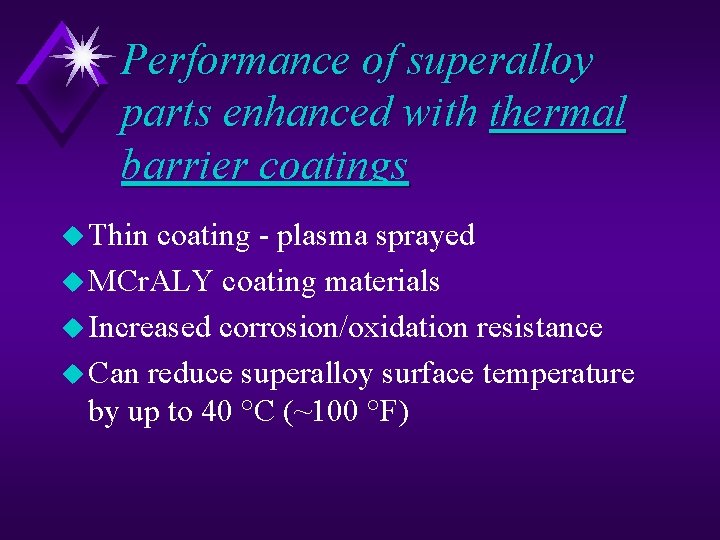
Performance of superalloy parts enhanced with thermal barrier coatings u Thin coating - plasma sprayed u MCr. ALY coating materials u Increased corrosion/oxidation resistance u Can reduce superalloy surface temperature by up to 40 °C (~100 °F)
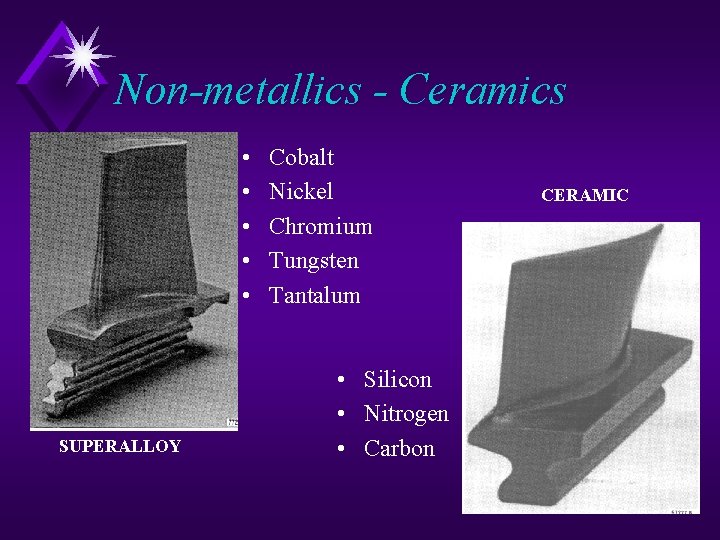
Non-metallics - Ceramics • • • SUPERALLOY Cobalt Nickel Chromium Tungsten Tantalum • Silicon • Nitrogen • Carbon CERAMIC
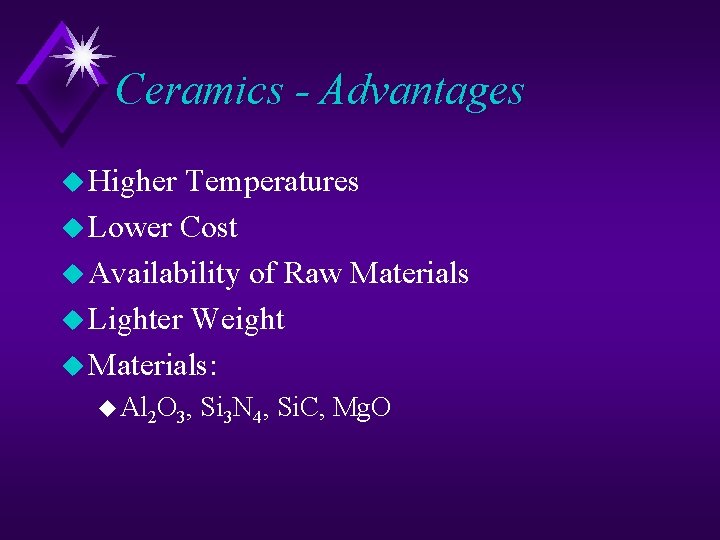
Ceramics - Advantages u Higher Temperatures u Lower Cost u Availability of Raw Materials u Lighter Weight u Materials: u Al 2 O 3, Si 3 N 4, Si. C, Mg. O
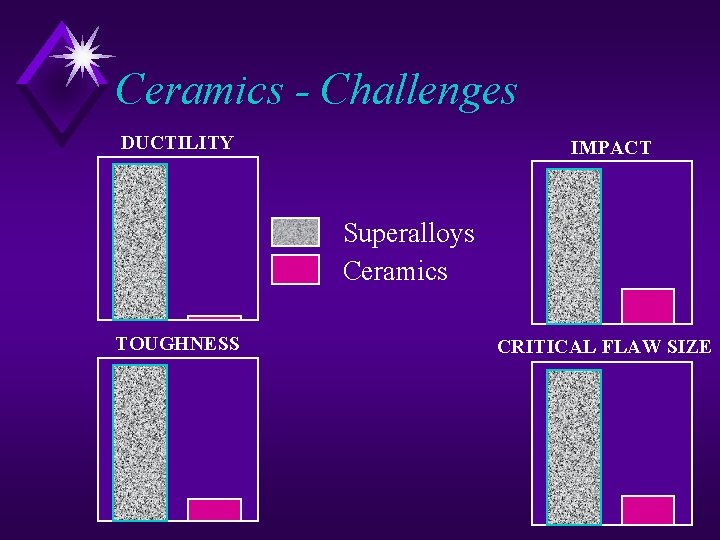
Ceramics - Challenges DUCTILITY IMPACT Superalloys Ceramics TOUGHNESS CRITICAL FLAW SIZE
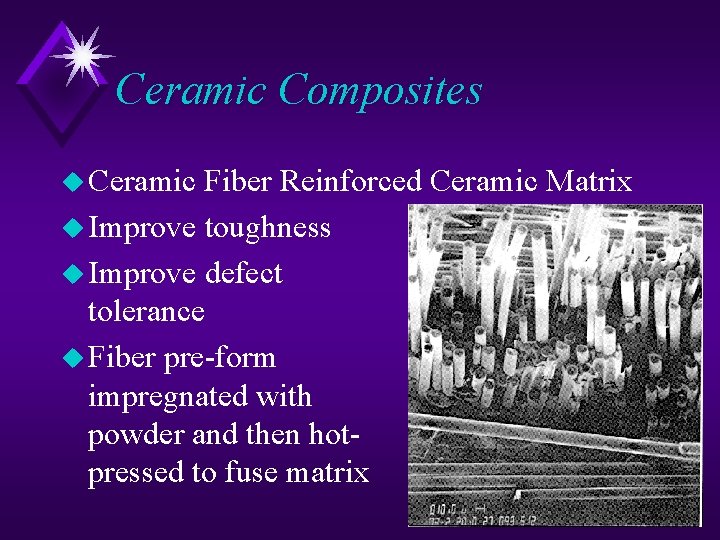
Ceramic Composites u Ceramic Fiber Reinforced Ceramic Matrix u Improve toughness u Improve defect tolerance u Fiber pre-form impregnated with powder and then hotpressed to fuse matrix
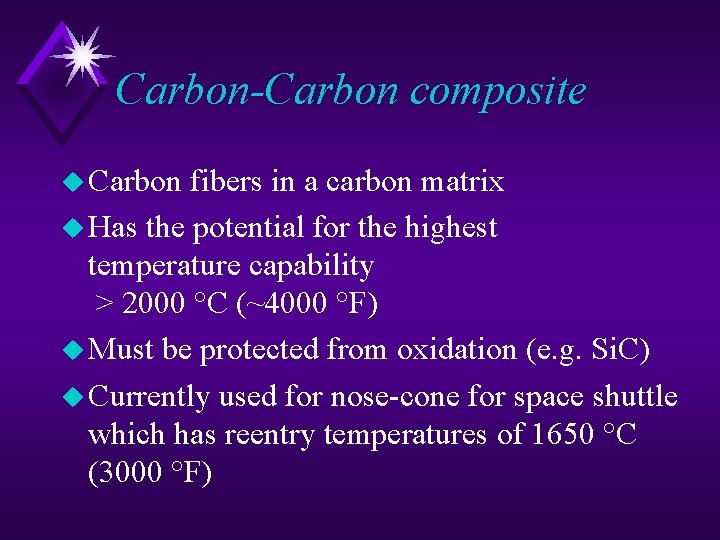
Carbon-Carbon composite u Carbon fibers in a carbon matrix u Has the potential for the highest temperature capability > 2000 °C (~4000 °F) u Must be protected from oxidation (e. g. Si. C) u Currently used for nose-cone for space shuttle which has reentry temperatures of 1650 °C (3000 °F)
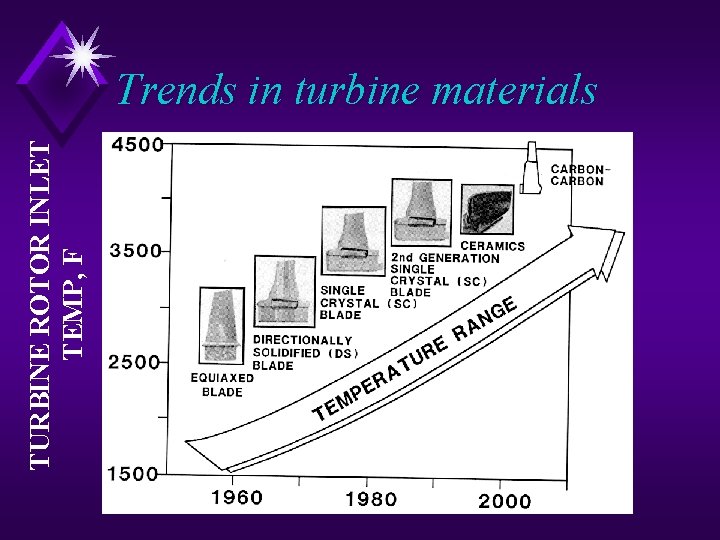
TURBINE ROTOR INLET TEMP, F Trends in turbine materials
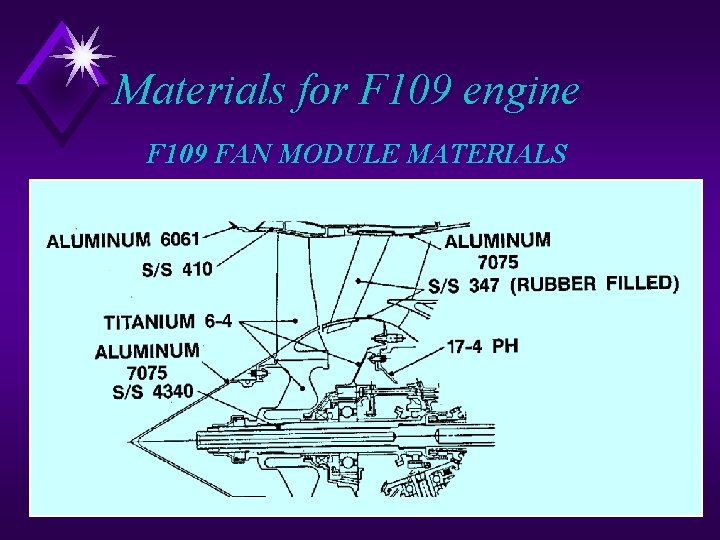
Materials for F 109 engine F 109 FAN MODULE MATERIALS
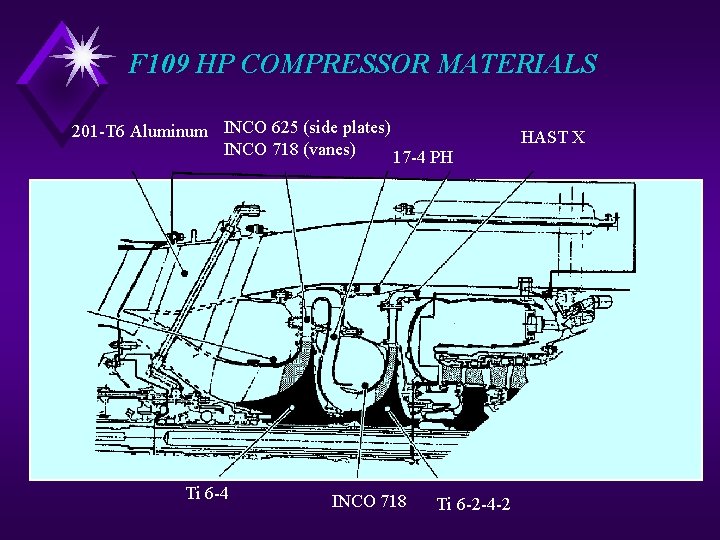
F 109 HP COMPRESSOR MATERIALS 201 -T 6 Aluminum INCO 625 (side plates) INCO 718 (vanes) 17 -4 PH Ti 6 -4 INCO 718 Ti 6 -2 -4 -2 HAST X
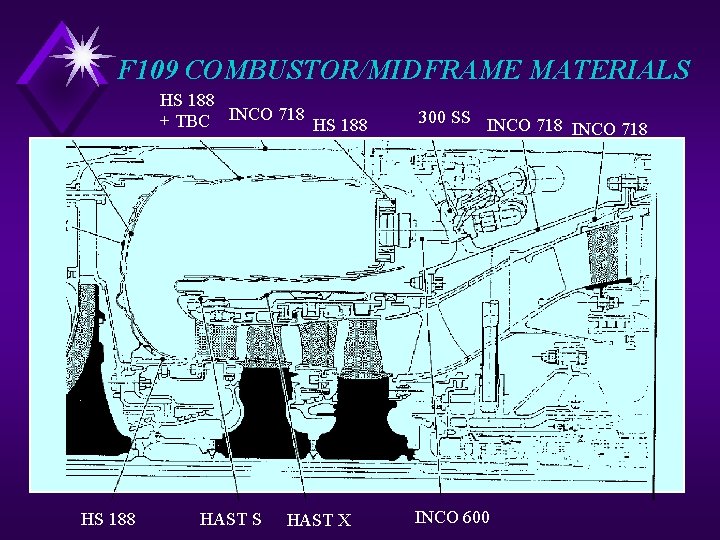
F 109 COMBUSTOR/MIDFRAME MATERIALS HS 188 + TBC INCO 718 HS 188 HAST S HAST X 300 SS INCO 718 INCO 600
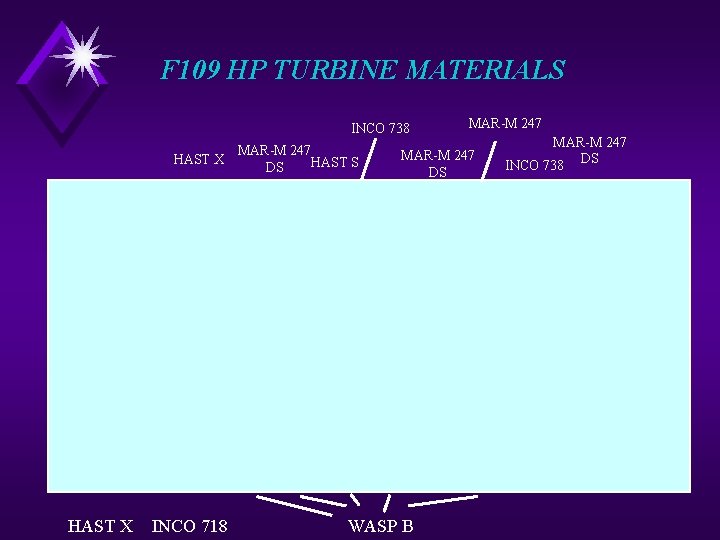
F 109 HP TURBINE MATERIALS INCO 738 HAST X INCO 718 MAR-M 247 HAST S DS MAR-M 247 DS WASP B MAR-M 247 INCO 738 DS
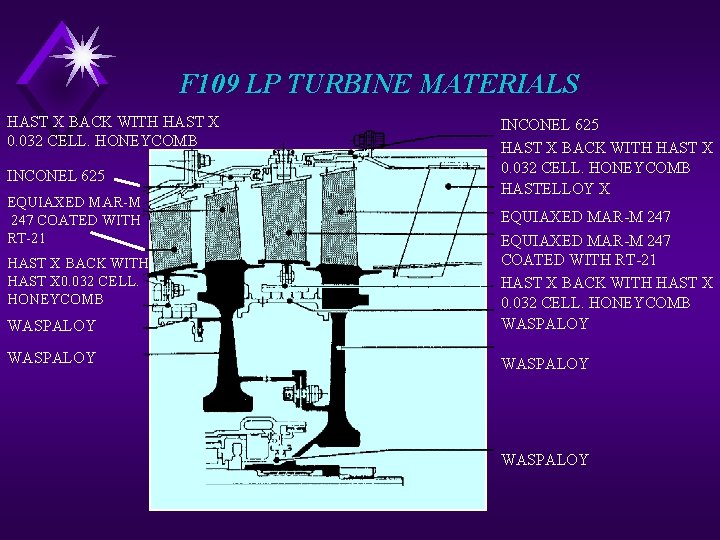
F 109 LP TURBINE MATERIALS HAST X BACK WITH HAST X 0. 032 CELL. HONEYCOMB INCONEL 625 EQUIAXED MAR-M 247 COATED WITH RT-21 INCONEL 625 HAST X BACK WITH HAST X 0. 032 CELL. HONEYCOMB HASTELLOY X WASPALOY EQUIAXED MAR-M 247 COATED WITH RT-21 HAST X BACK WITH HAST X 0. 032 CELL. HONEYCOMB WASPALOY HAST X BACK WITH HAST X 0. 032 CELL. HONEYCOMB WASPALOY
Yelvington jet aviation aircraft fuel
The fan blade is speeding up. what are the signs of
Quick find algorithm
Divergent duct jet engine
How does a jet engine work animation
Jet engine
Clamshell thrust reverser
Propulsion efficiency
External vs internal combustion engine
Direct materials budget with multiple materials
Materials that is useful and harmful
Man made materials
Adapting and adopting materials
Favourite cars
Các môn thể thao bắt đầu bằng tiếng nhảy
Sự nuôi và dạy con của hươu
điện thế nghỉ
Dạng đột biến một nhiễm là
Nguyên nhân của sự mỏi cơ sinh 8
Trời xanh đây là của chúng ta thể thơ
Chó sói
Thiếu nhi thế giới liên hoan
Fecboak
Các châu lục và đại dương trên thế giới
Một số thể thơ truyền thống
Thế nào là hệ số cao nhất
Hệ hô hấp
Tư thế ngồi viết
So nguyen to
Hình ảnh bộ gõ cơ thể búng tay
đặc điểm cơ thể của người tối cổ
Mật thư anh em như thể tay chân
Tư thế worm breton là gì