Future prospects of semiconductor materials for solar and
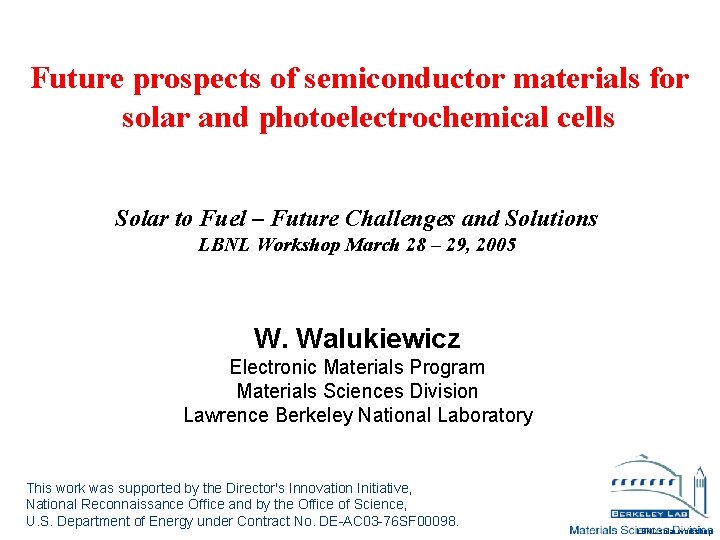
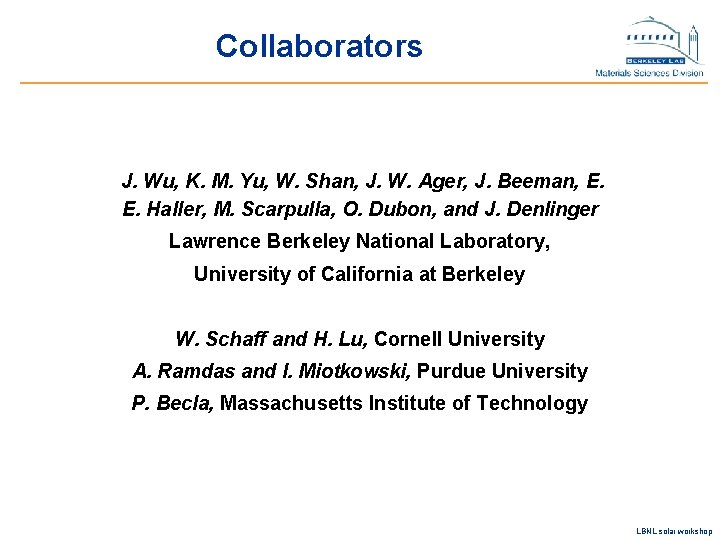
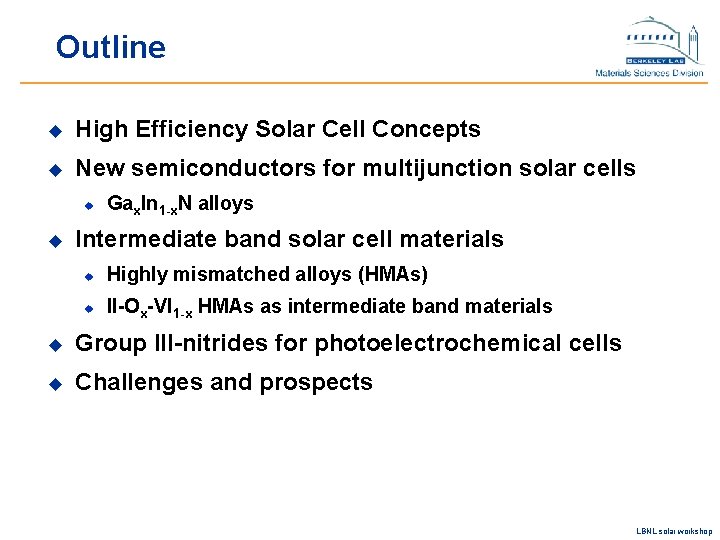
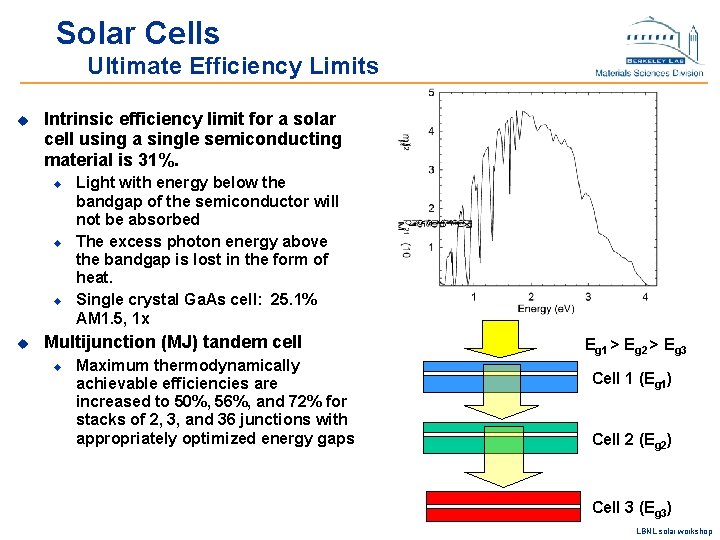
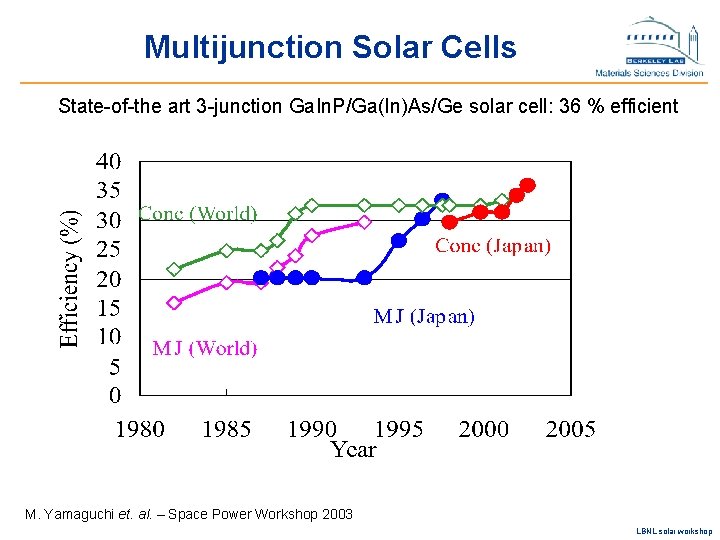
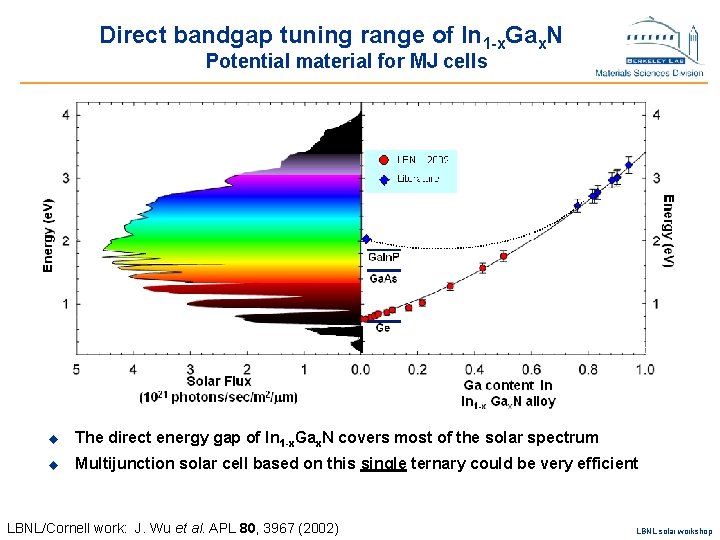
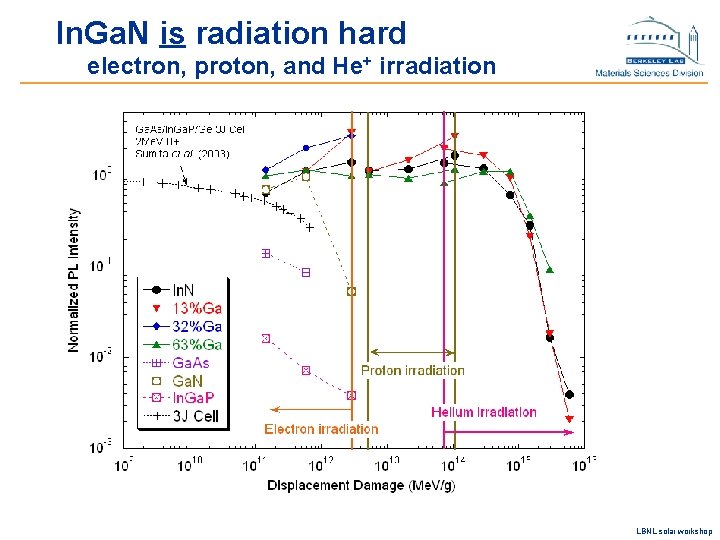
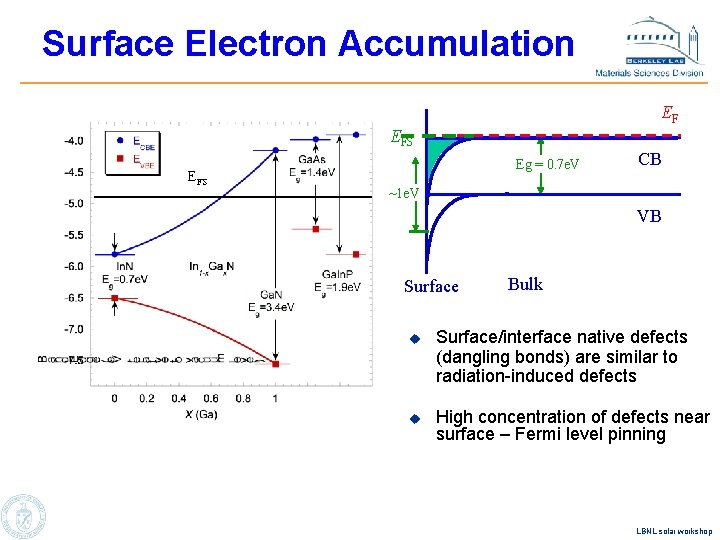
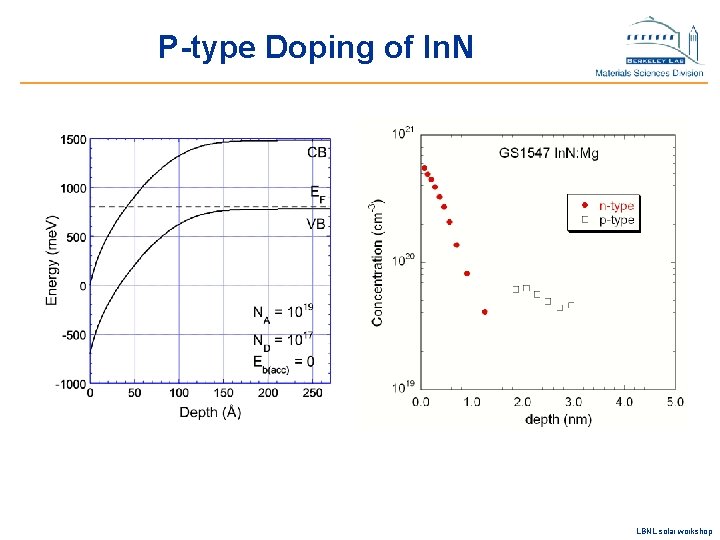
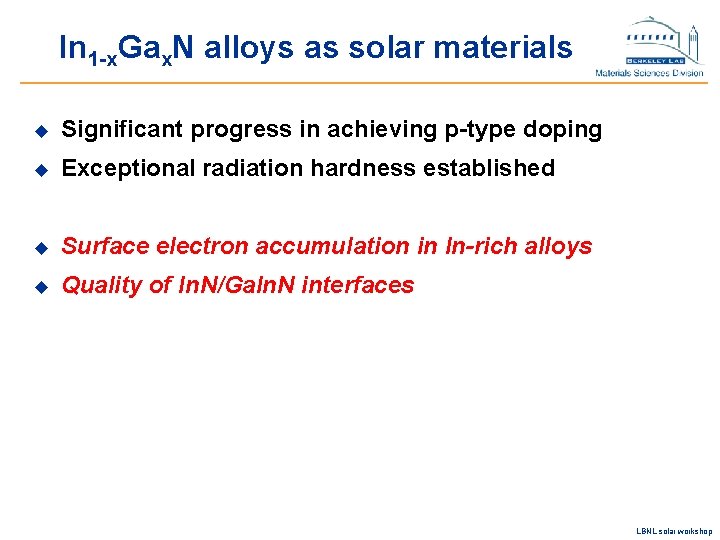
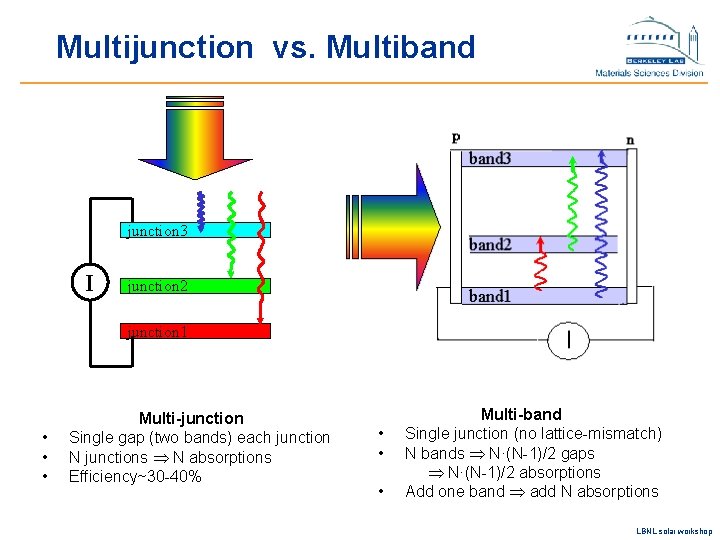
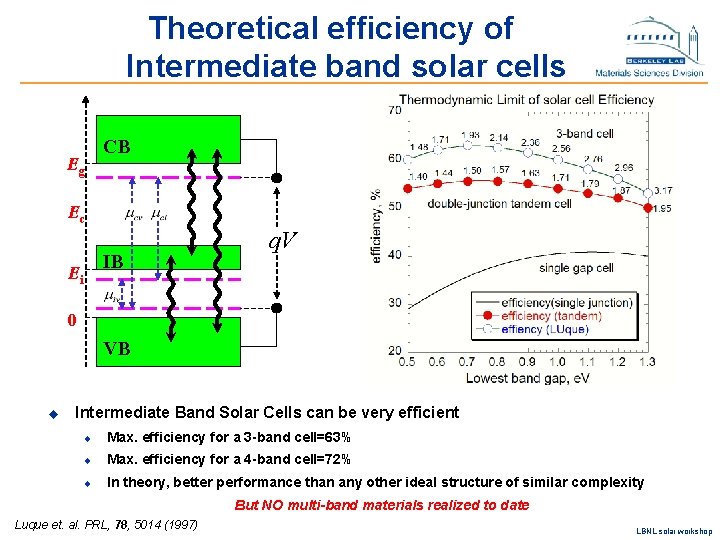
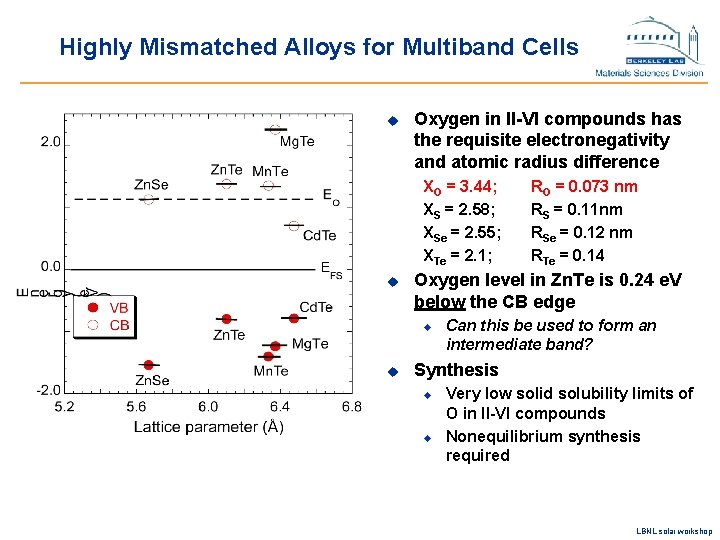
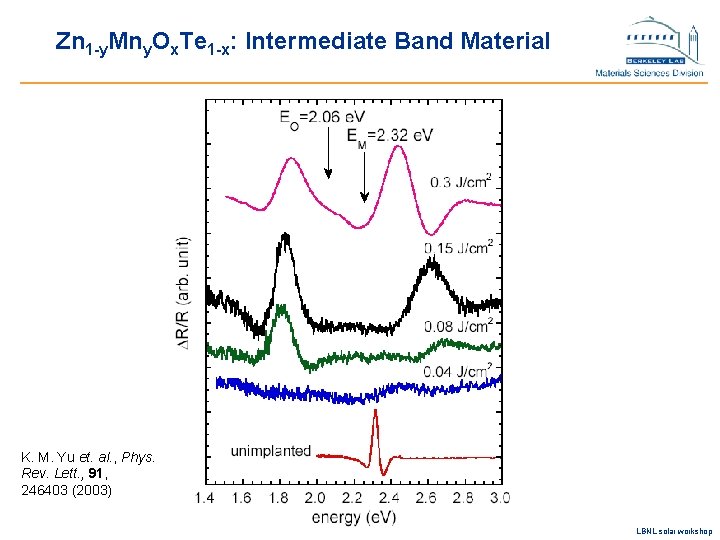
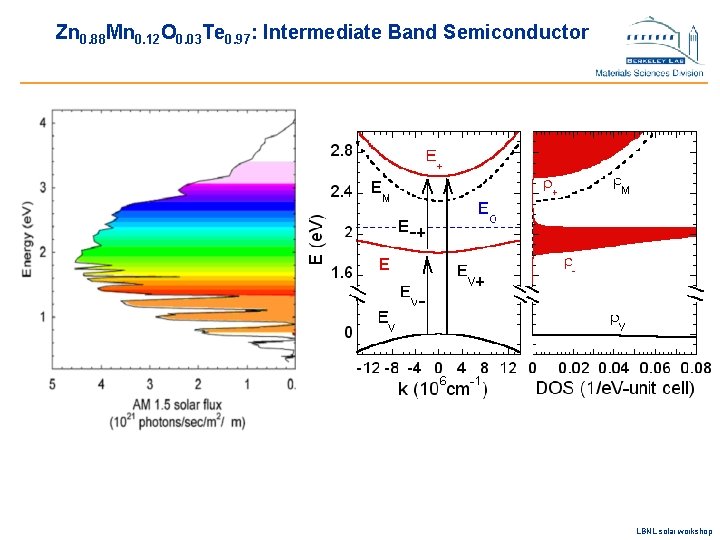
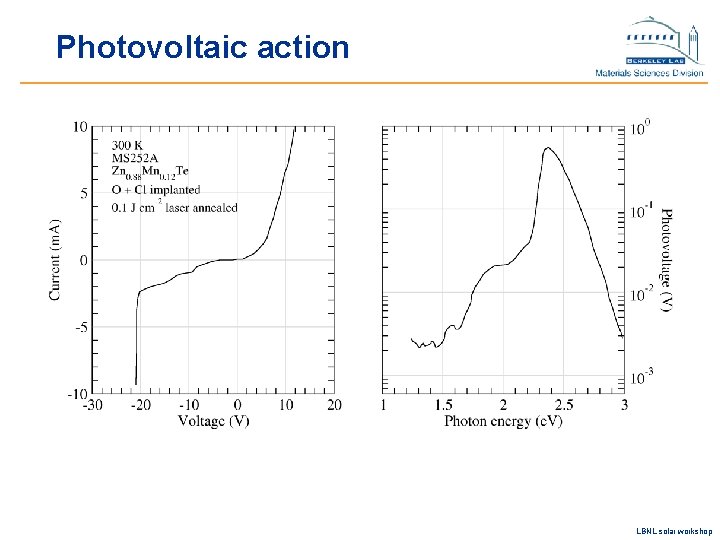
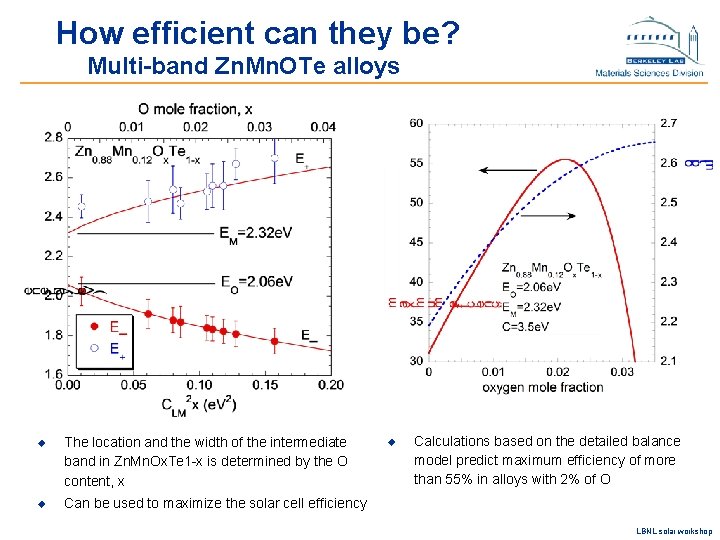
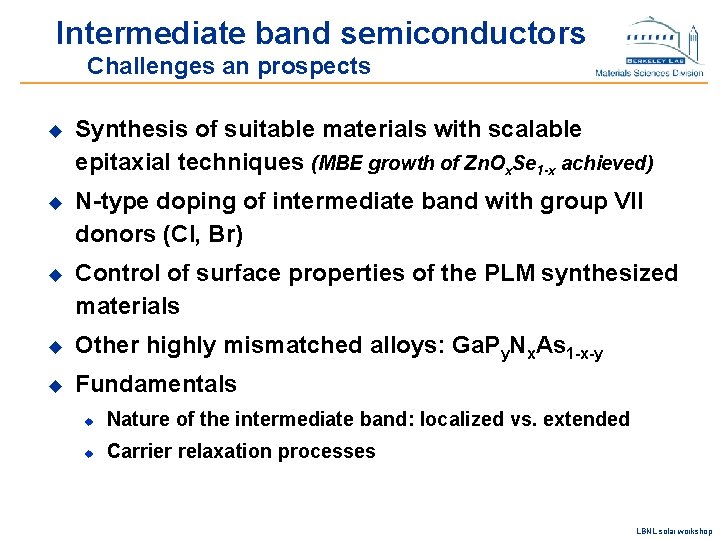
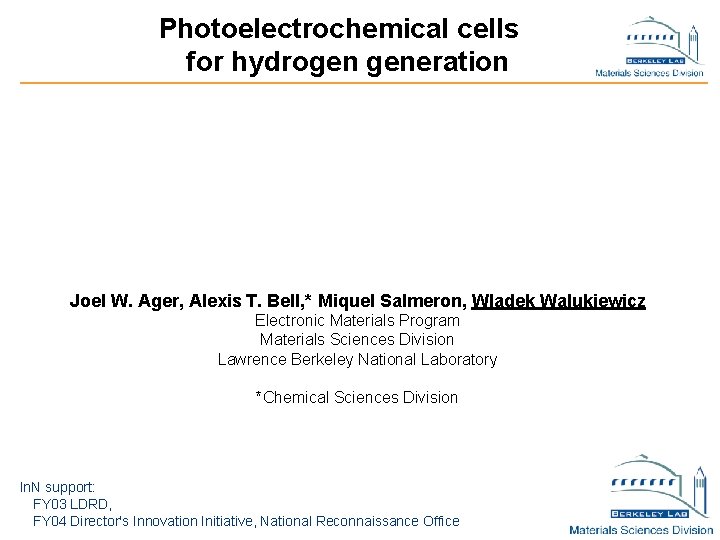
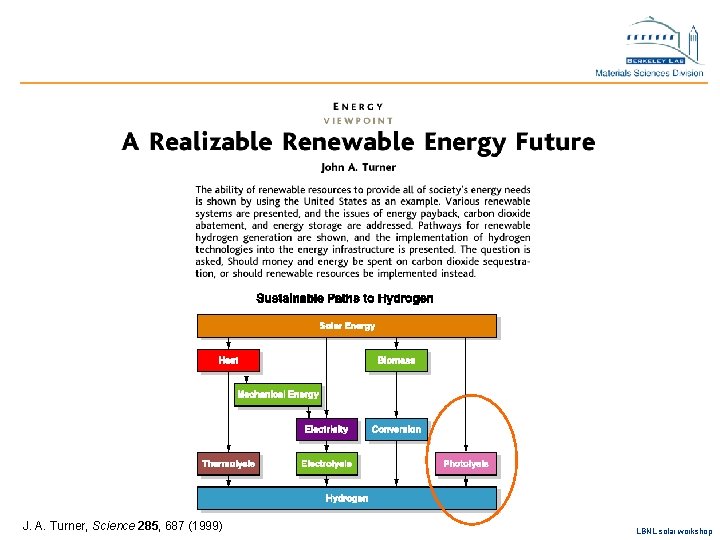
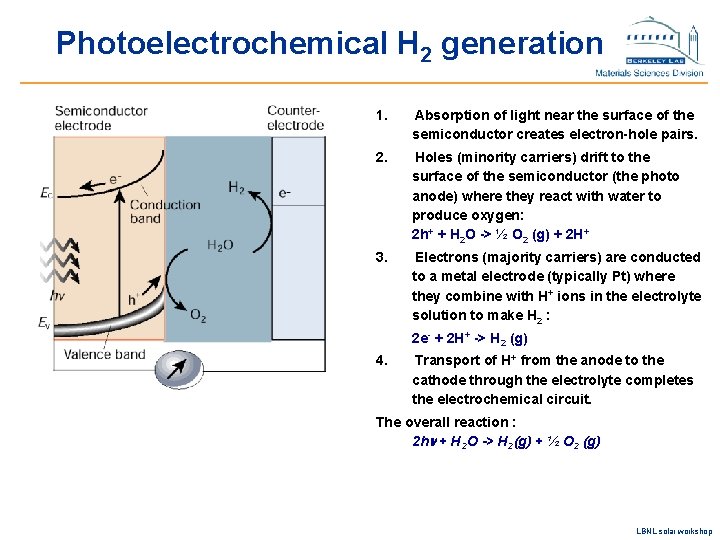
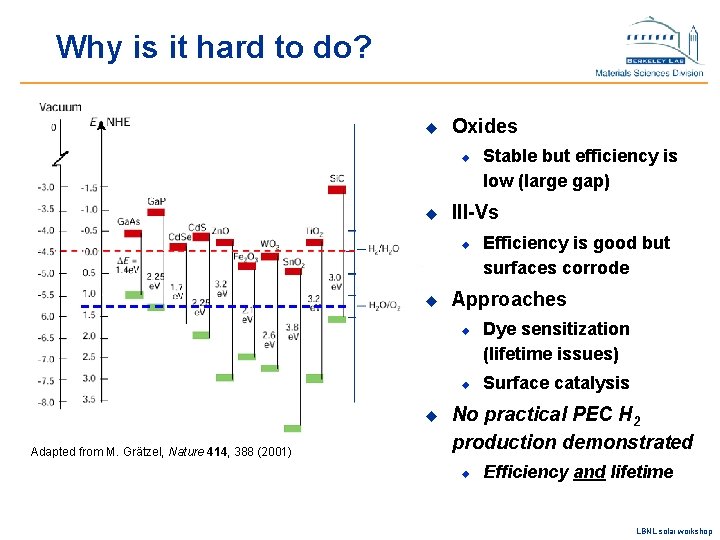
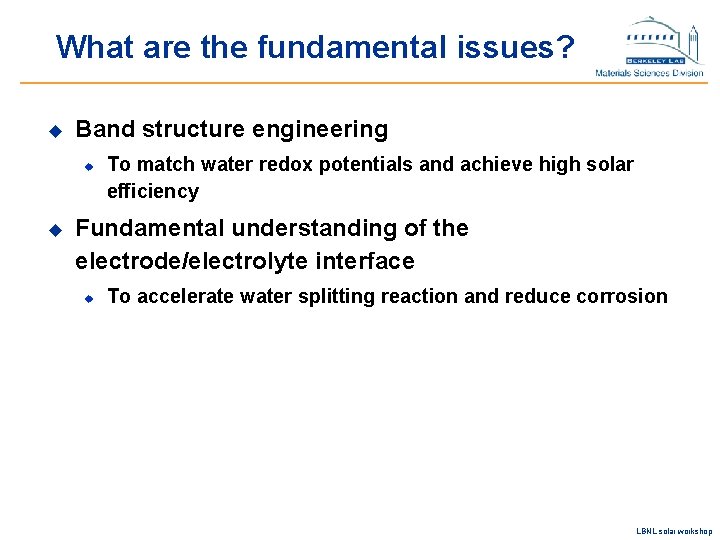
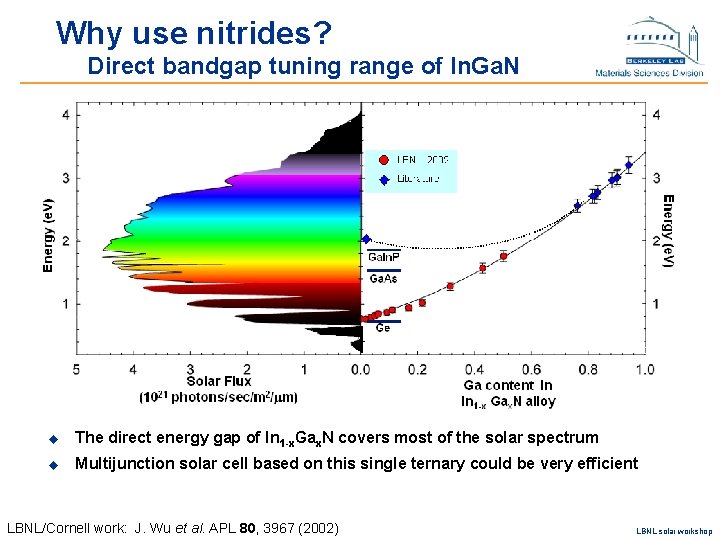
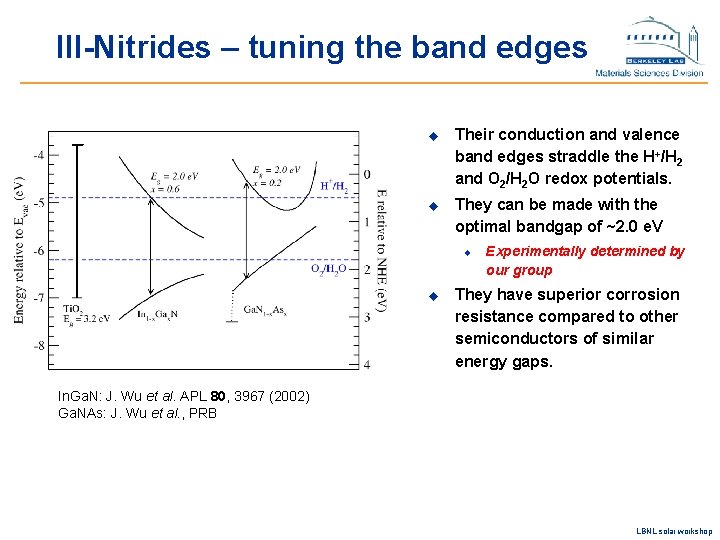
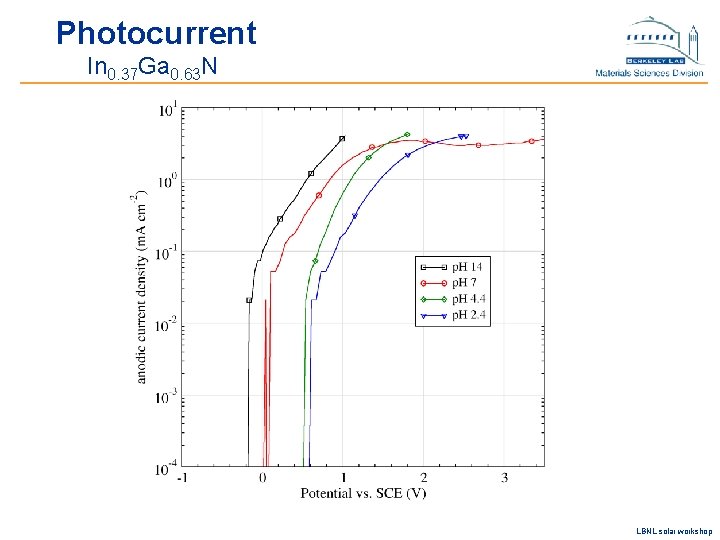
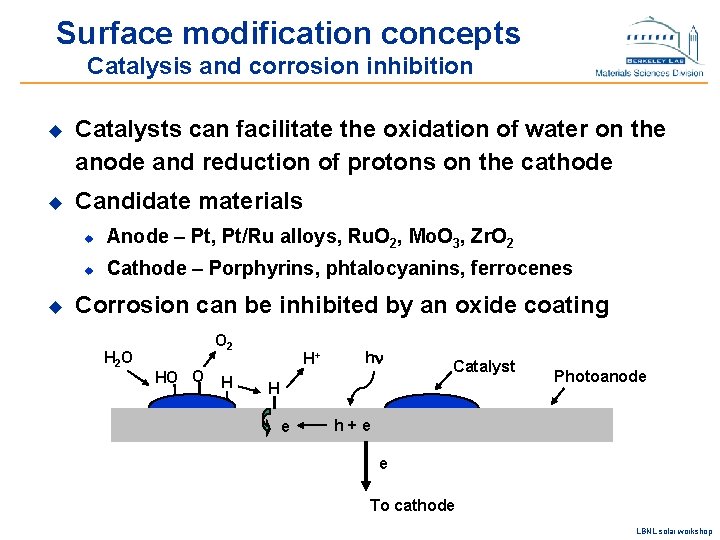
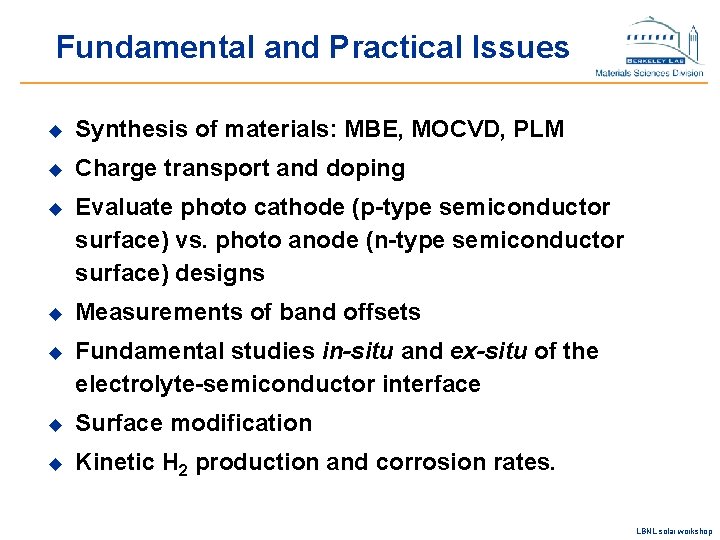
- Slides: 28
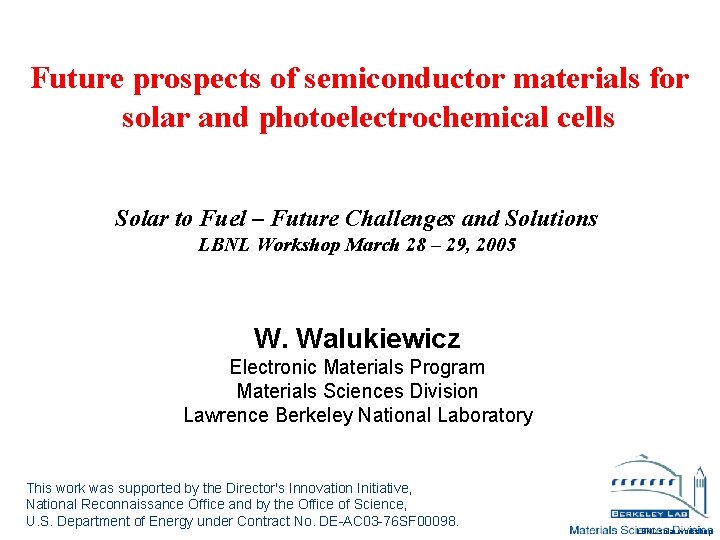
Future prospects of semiconductor materials for solar and photoelectrochemical cells Solar to Fuel – Future Challenges and Solutions LBNL Workshop March 28 – 29, 2005 W. Walukiewicz Electronic Materials Program Materials Sciences Division Lawrence Berkeley National Laboratory This work was supported by the Director's Innovation Initiative, National Reconnaissance Office and by the Office of Science, U. S. Department of Energy under Contract No. DE-AC 03 -76 SF 00098. LBNL solar workshop
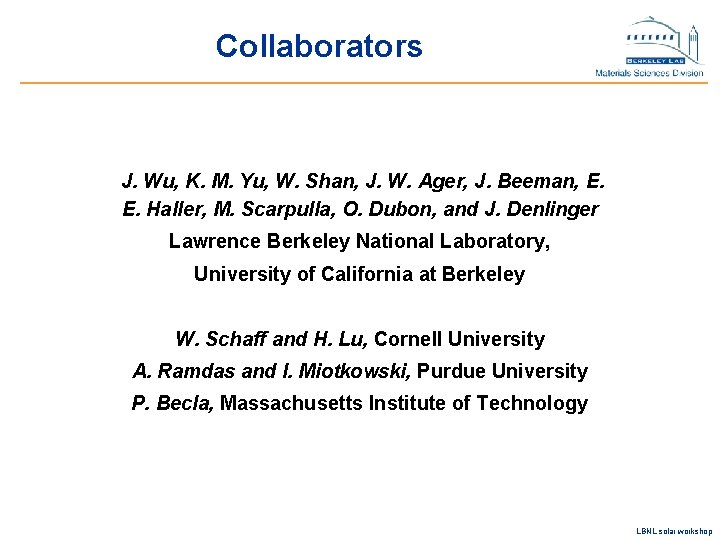
Collaborators J. Wu, K. M. Yu, W. Shan, J. W. Ager, J. Beeman, E. E. Haller, M. Scarpulla, O. Dubon, and J. Denlinger Lawrence Berkeley National Laboratory, University of California at Berkeley W. Schaff and H. Lu, Cornell University A. Ramdas and I. Miotkowski, Purdue University P. Becla, Massachusetts Institute of Technology LBNL solar workshop
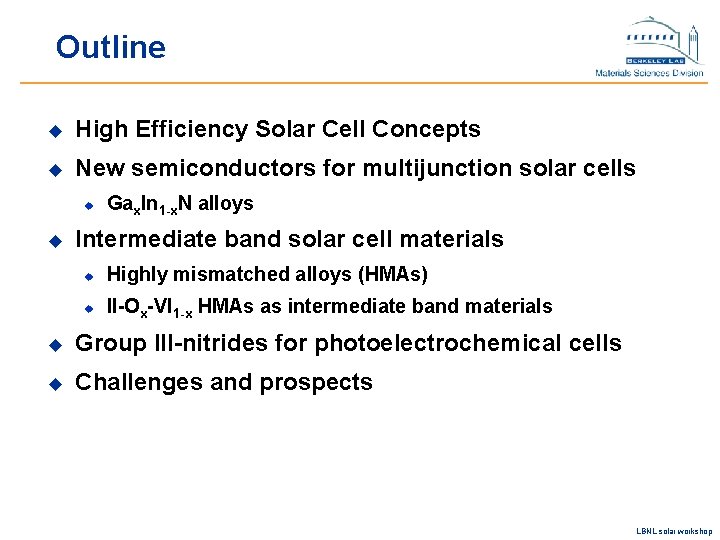
Outline u High Efficiency Solar Cell Concepts u New semiconductors for multijunction solar cells u u Gax. In 1 -x. N alloys Intermediate band solar cell materials u Highly mismatched alloys (HMAs) u II-Ox-VI 1 -x HMAs as intermediate band materials u Group III-nitrides for photoelectrochemical cells u Challenges and prospects LBNL solar workshop
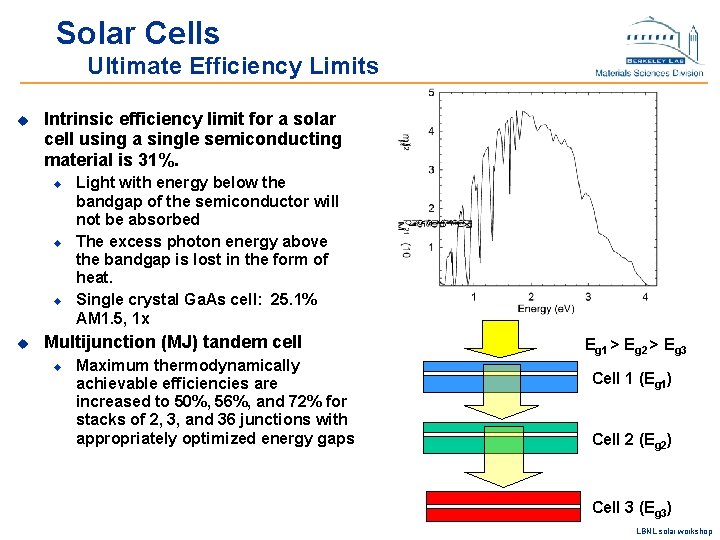
Solar Cells Ultimate Efficiency Limits u Intrinsic efficiency limit for a solar cell using a single semiconducting material is 31%. u u Light with energy below the bandgap of the semiconductor will not be absorbed The excess photon energy above the bandgap is lost in the form of heat. Single crystal Ga. As cell: 25. 1% AM 1. 5, 1 x Multijunction (MJ) tandem cell u Maximum thermodynamically achievable efficiencies are increased to 50%, 56%, and 72% for stacks of 2, 3, and 36 junctions with appropriately optimized energy gaps Eg 1 > Eg 2 > Eg 3 Cell 1 (Eg 1) Cell 2 (Eg 2) Cell 3 (Eg 3) LBNL solar workshop
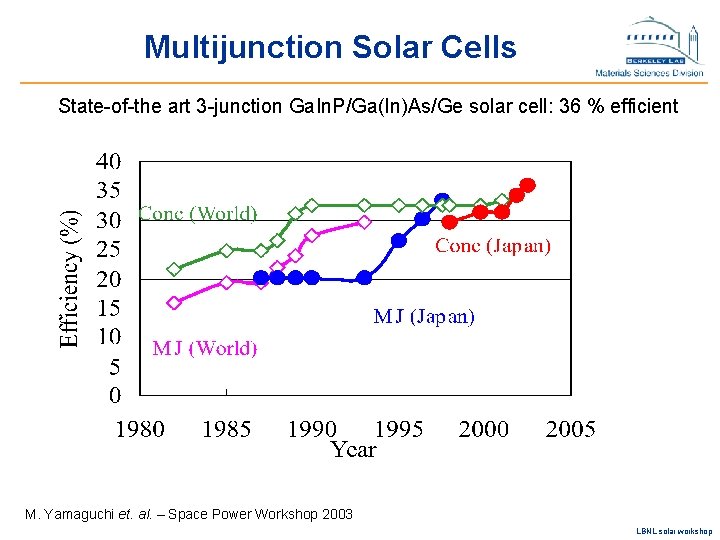
Multijunction Solar Cells State-of-the art 3 -junction Ga. In. P/Ga(In)As/Ge solar cell: 36 % efficient M. Yamaguchi et. al. – Space Power Workshop 2003 LBNL solar workshop
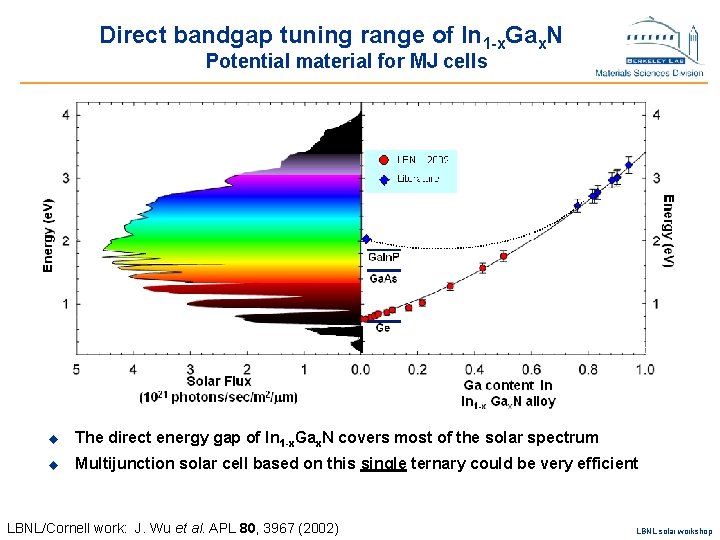
Direct bandgap tuning range of In 1 -x. Gax. N Potential material for MJ cells u The direct energy gap of In 1 -x. Gax. N covers most of the solar spectrum u Multijunction solar cell based on this single ternary could be very efficient LBNL/Cornell work: J. Wu et al. APL 80, 3967 (2002) LBNL solar workshop
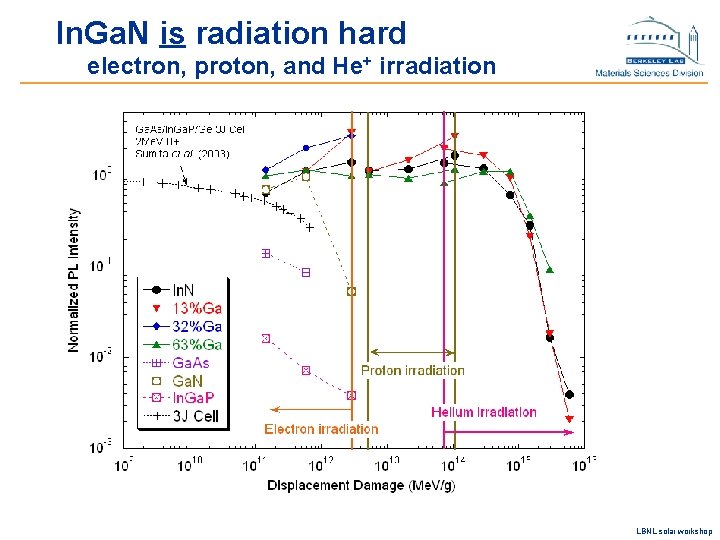
In. Ga. N is radiation hard electron, proton, and He+ irradiation LBNL solar workshop
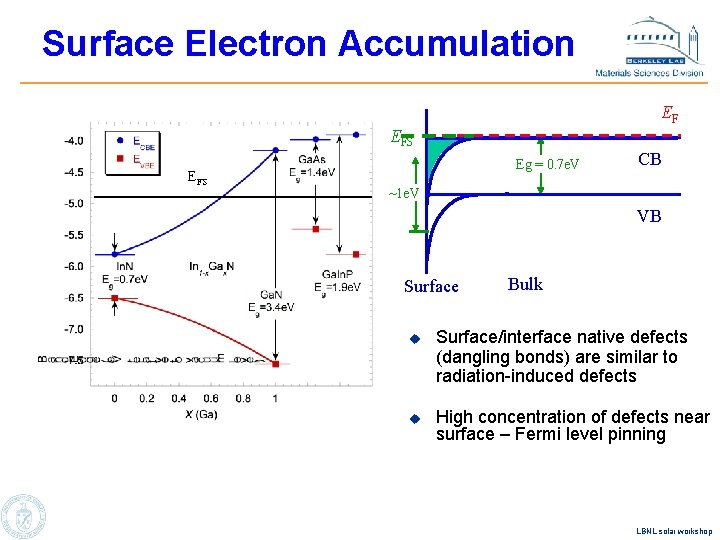
Surface Electron Accumulation EF EFS Eg = 0. 7 e. V CB ~1 e. V VB Surface Bulk u Surface/interface native defects (dangling bonds) are similar to radiation-induced defects u High concentration of defects near surface – Fermi level pinning LBNL solar workshop
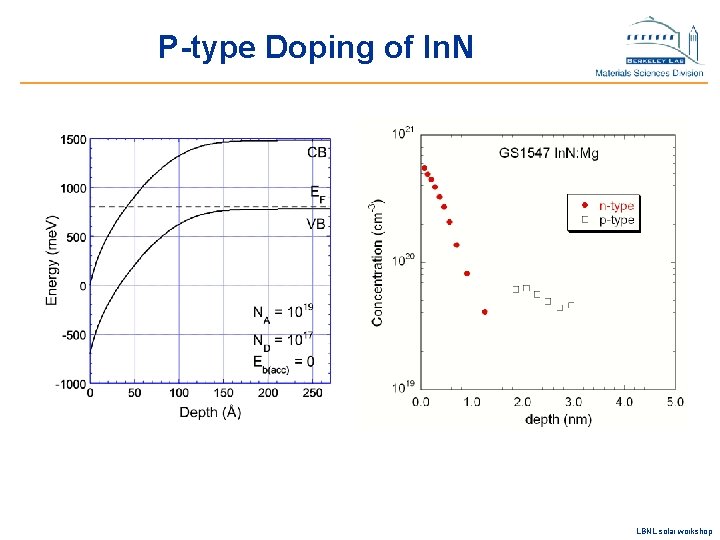
P-type Doping of In. N LBNL solar workshop
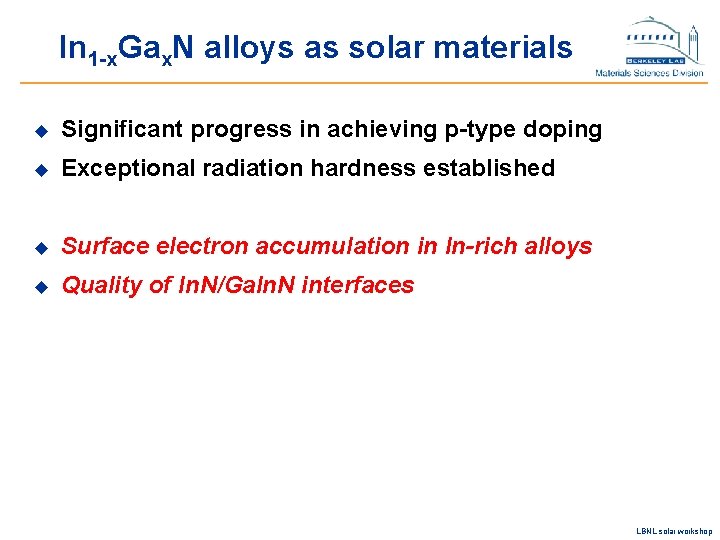
In 1 -x. Gax. N alloys as solar materials u Significant progress in achieving p-type doping u Exceptional radiation hardness established u Surface electron accumulation in In-rich alloys u Quality of In. N/Ga. In. N interfaces LBNL solar workshop
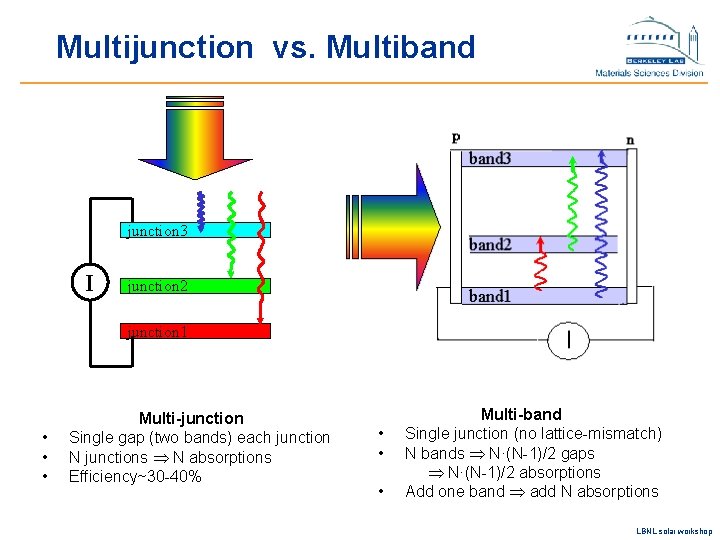
Multijunction vs. Multiband junction 3 I junction 2 junction 1 • • • Multi-junction Single gap (two bands) each junction N junctions N absorptions Efficiency~30 -40% • • • Multi-band Single junction (no lattice-mismatch) N bands N·(N-1)/2 gaps N·(N-1)/2 absorptions Add one band add N absorptions LBNL solar workshop
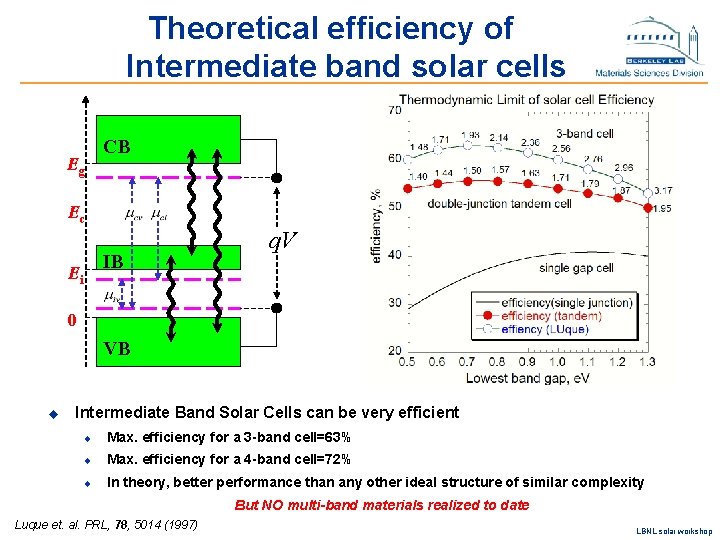
Theoretical efficiency of Intermediate band solar cells Eg CB Ec IB Ei q. V 0 VB u Intermediate Band Solar Cells can be very efficient u Max. efficiency for a 3 -band cell=63% u Max. efficiency for a 4 -band cell=72% u In theory, better performance than any other ideal structure of similar complexity But NO multi-band materials realized to date Luque et. al. PRL, 78, 5014 (1997) LBNL solar workshop
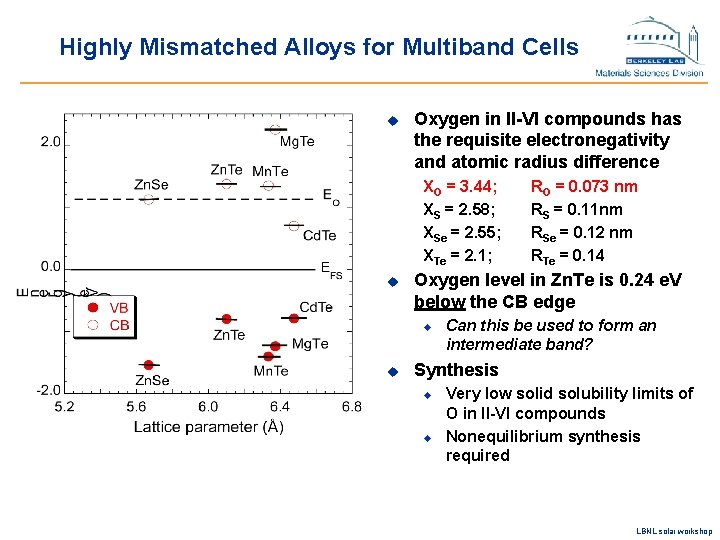
Highly Mismatched Alloys for Multiband Cells u Oxygen in II-VI compounds has the requisite electronegativity and atomic radius difference XO = 3. 44; XS = 2. 58; XSe = 2. 55; XTe = 2. 1; u Oxygen level in Zn. Te is 0. 24 e. V below the CB edge u u RO = 0. 073 nm RS = 0. 11 nm RSe = 0. 12 nm RTe = 0. 14 Can this be used to form an intermediate band? Synthesis u u Very low solid solubility limits of O in II-VI compounds Nonequilibrium synthesis required LBNL solar workshop
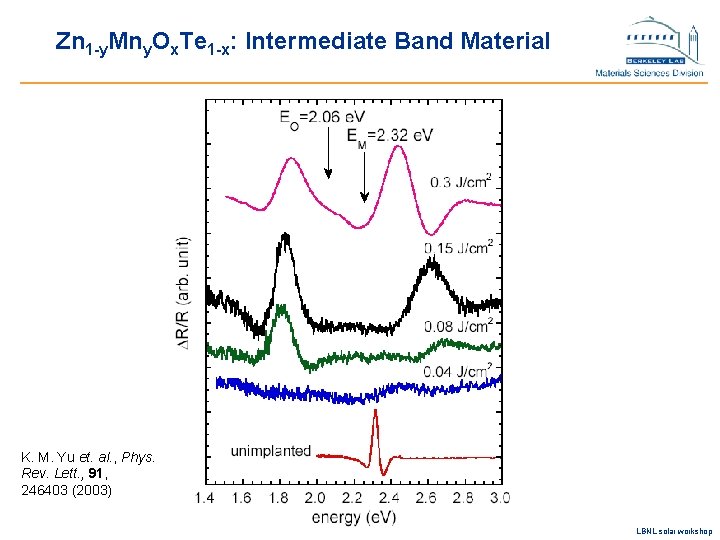
Zn 1 -y. Mny. Ox. Te 1 -x: Intermediate Band Material K. M. Yu et. al. , Phys. Rev. Lett. , 91, 246403 (2003) LBNL solar workshop
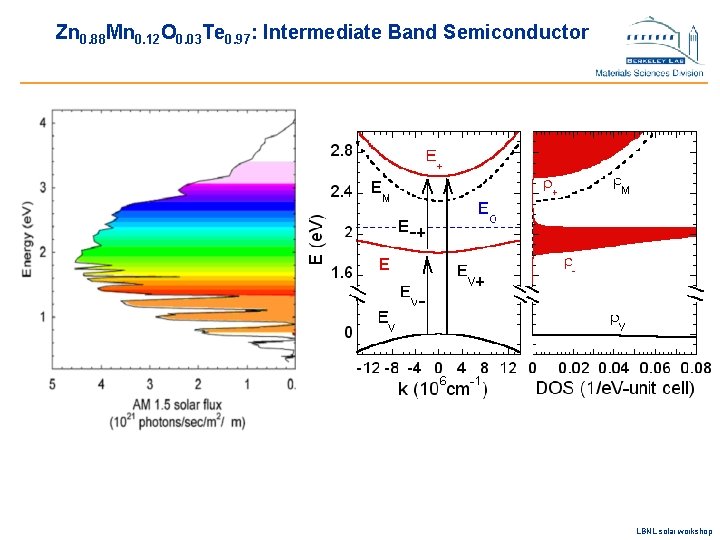
Zn 0. 88 Mn 0. 12 O 0. 03 Te 0. 97: Intermediate Band Semiconductor LBNL solar workshop
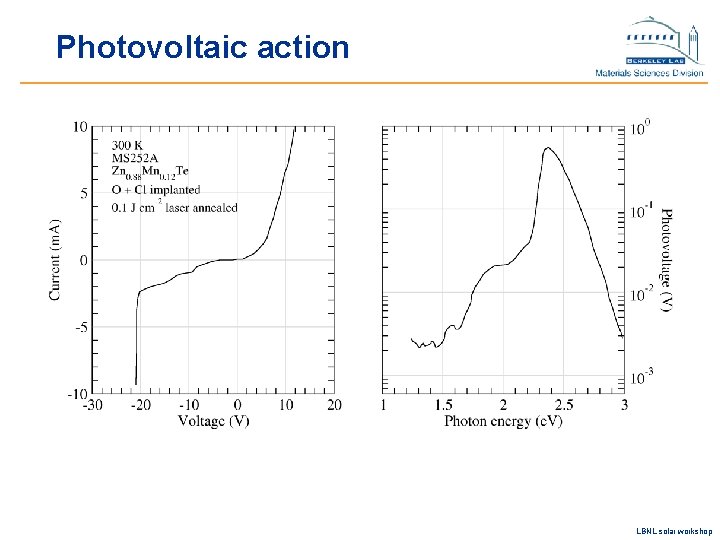
Photovoltaic action LBNL solar workshop
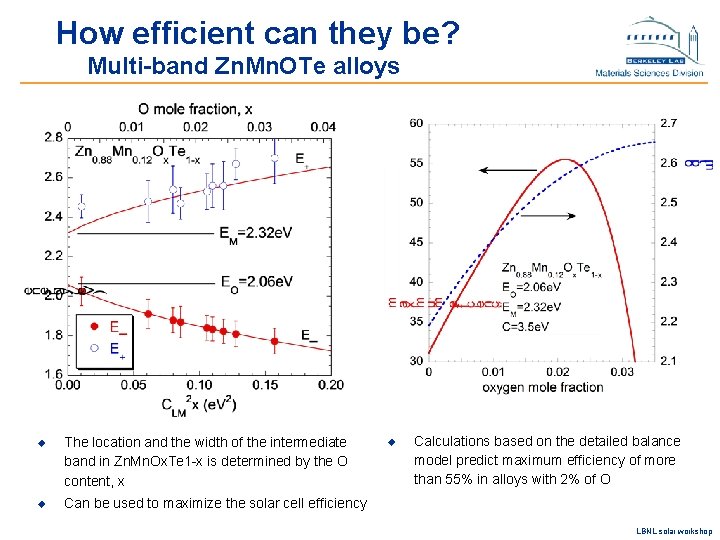
How efficient can they be? Multi-band Zn. Mn. OTe alloys u The location and the width of the intermediate band in Zn. Mn. Ox. Te 1 -x is determined by the O content, x u Can be used to maximize the solar cell efficiency u Calculations based on the detailed balance model predict maximum efficiency of more than 55% in alloys with 2% of O LBNL solar workshop
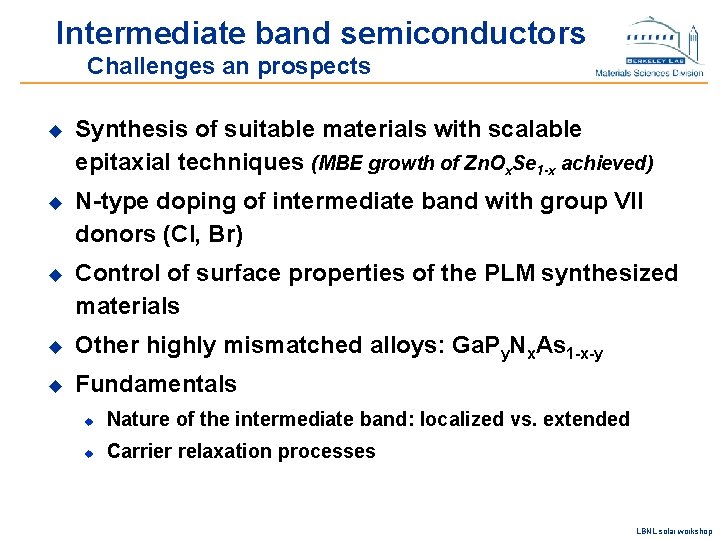
Intermediate band semiconductors Challenges an prospects u Synthesis of suitable materials with scalable epitaxial techniques (MBE growth of Zn. Ox. Se 1 -x achieved) u N-type doping of intermediate band with group VII donors (Cl, Br) u Control of surface properties of the PLM synthesized materials u Other highly mismatched alloys: Ga. Py. Nx. As 1 -x-y u Fundamentals u Nature of the intermediate band: localized vs. extended u Carrier relaxation processes LBNL solar workshop
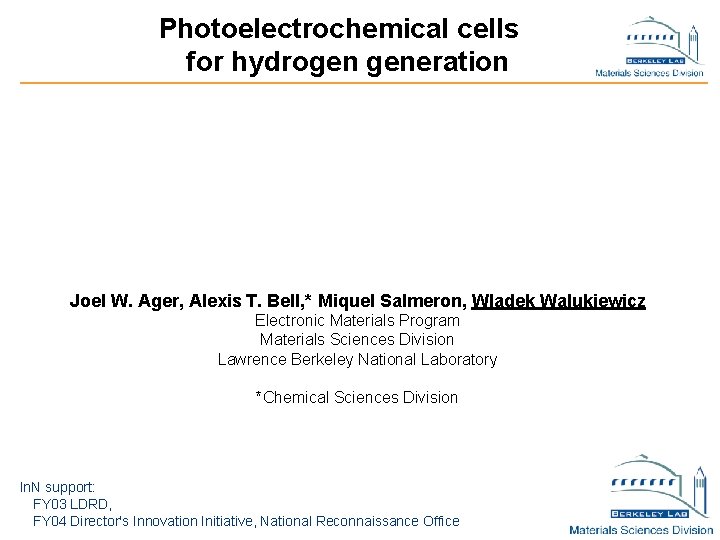
Photoelectrochemical cells for hydrogen generation Joel W. Ager, Alexis T. Bell, * Miquel Salmeron, Wladek Walukiewicz Electronic Materials Program Materials Sciences Division Lawrence Berkeley National Laboratory *Chemical Sciences Division In. N support: FY 03 LDRD, FY 04 Director's Innovation Initiative, National Reconnaissance Office
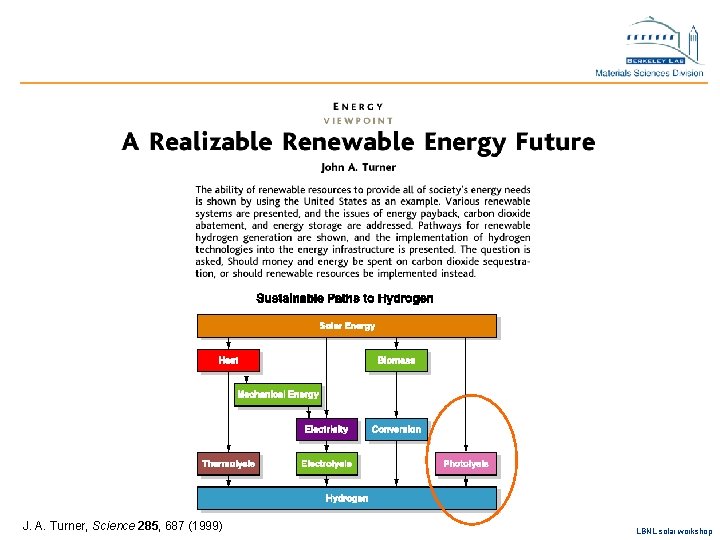
J. A. Turner, Science 285, 687 (1999) LBNL solar workshop
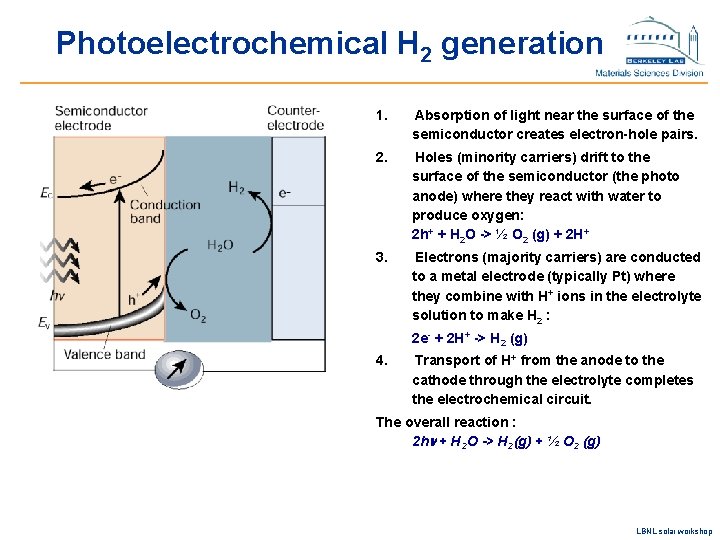
Photoelectrochemical H 2 generation 1. Absorption of light near the surface of the semiconductor creates electron-hole pairs. 2. Holes (minority carriers) drift to the surface of the semiconductor (the photo anode) where they react with water to produce oxygen: 2 h+ + H 2 O -> ½ O 2 (g) + 2 H+ 3. Electrons (majority carriers) are conducted to a metal electrode (typically Pt) where they combine with H+ ions in the electrolyte solution to make H 2 : 2 e- + 2 H+ -> H 2 (g) 4. Transport of H+ from the anode to the cathode through the electrolyte completes the electrochemical circuit. The overall reaction : 2 hn + H 2 O -> H 2(g) + ½ O 2 (g) LBNL solar workshop
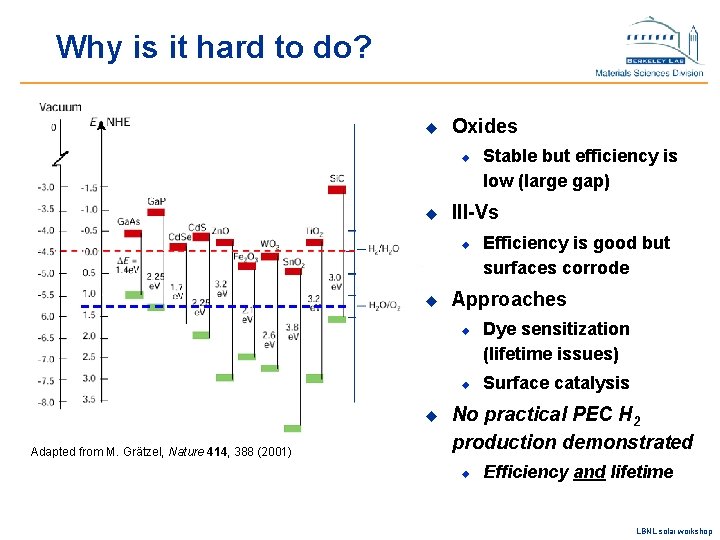
Why is it hard to do? u Oxides u u III-Vs u u u Adapted from M. Grätzel, Nature 414, 388 (2001) Efficiency is good but surfaces corrode Approaches u u Stable but efficiency is low (large gap) Dye sensitization (lifetime issues) Surface catalysis No practical PEC H 2 production demonstrated u Efficiency and lifetime LBNL solar workshop
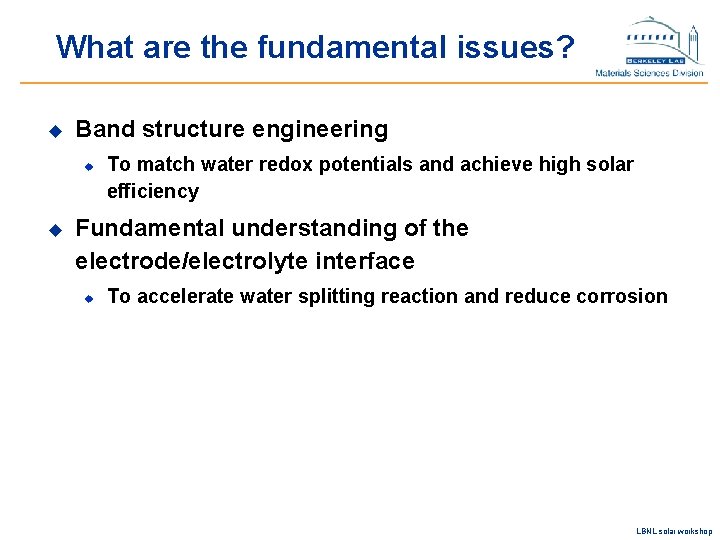
What are the fundamental issues? u Band structure engineering u u To match water redox potentials and achieve high solar efficiency Fundamental understanding of the electrode/electrolyte interface u To accelerate water splitting reaction and reduce corrosion LBNL solar workshop
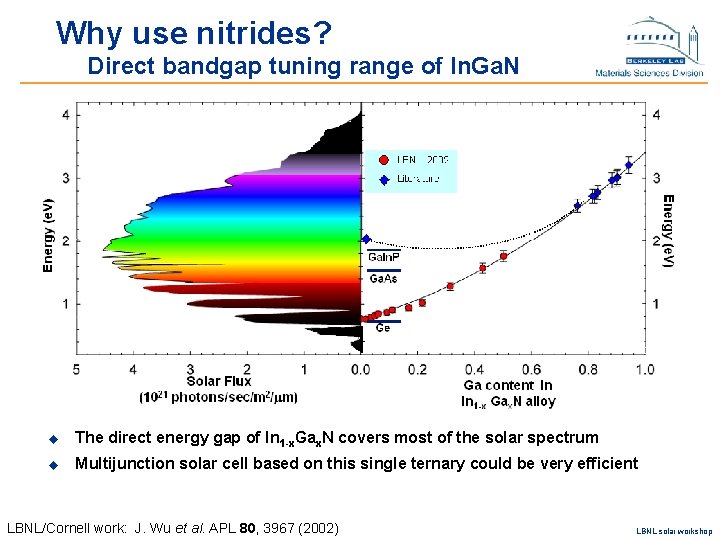
Why use nitrides? Direct bandgap tuning range of In. Ga. N u The direct energy gap of In 1 -x. Gax. N covers most of the solar spectrum u Multijunction solar cell based on this single ternary could be very efficient LBNL/Cornell work: J. Wu et al. APL 80, 3967 (2002) LBNL solar workshop
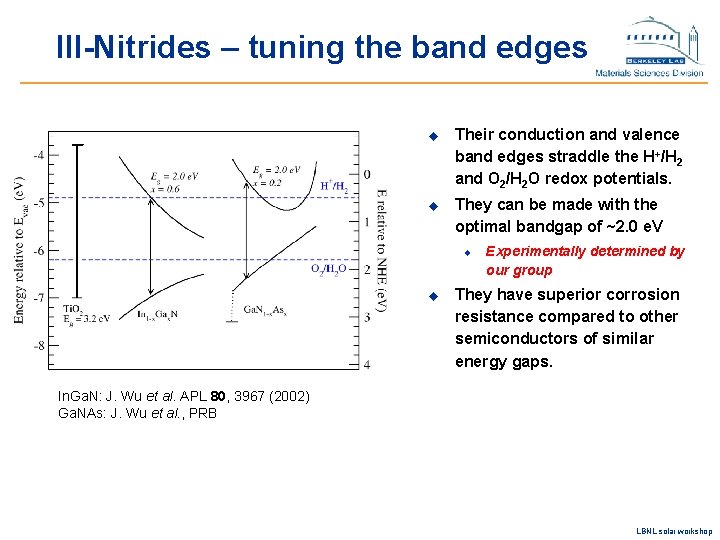
III-Nitrides – tuning the band edges u Their conduction and valence band edges straddle the H+/H 2 and O 2/H 2 O redox potentials. u They can be made with the optimal bandgap of ~2. 0 e. V u u Experimentally determined by our group They have superior corrosion resistance compared to other semiconductors of similar energy gaps. In. Ga. N: J. Wu et al. APL 80, 3967 (2002) Ga. NAs: J. Wu et al. , PRB LBNL solar workshop
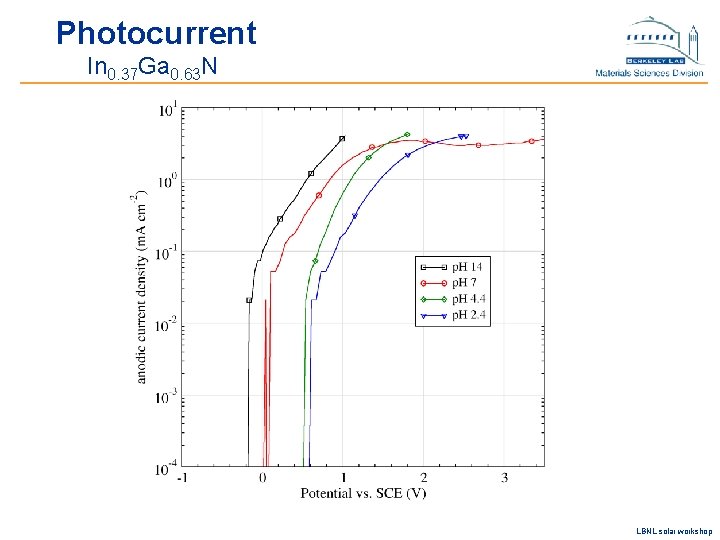
Photocurrent In 0. 37 Ga 0. 63 N LBNL solar workshop
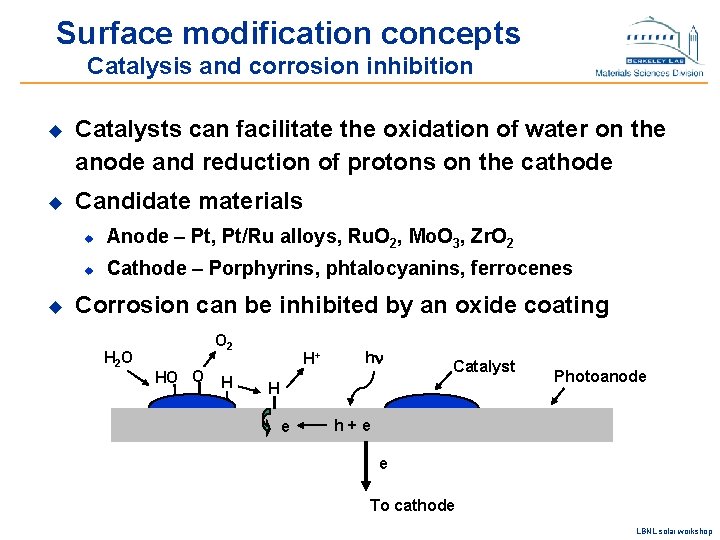
Surface modification concepts Catalysis and corrosion inhibition u Catalysts can facilitate the oxidation of water on the anode and reduction of protons on the cathode u Candidate materials u u Anode – Pt, Pt/Ru alloys, Ru. O 2, Mo. O 3, Zr. O 2 u Cathode – Porphyrins, phtalocyanins, ferrocenes Corrosion can be inhibited by an oxide coating H 2 O O 2 HO O H H+ hn Catalyst H e Photoanode h+e e To cathode LBNL solar workshop
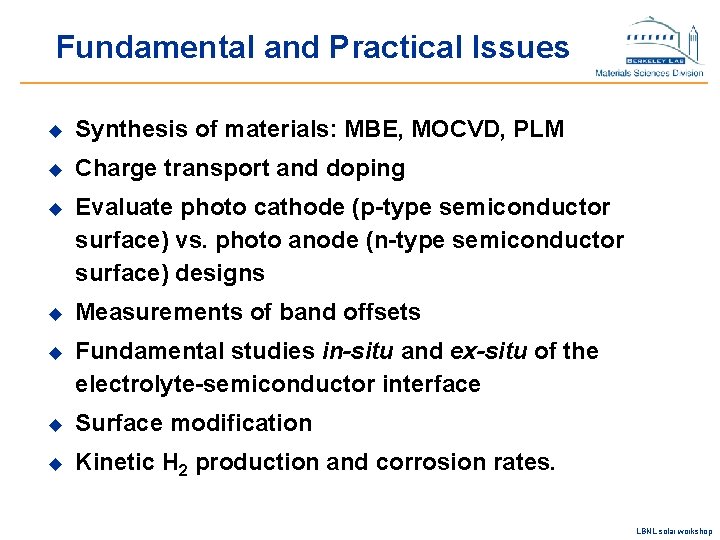
Fundamental and Practical Issues u Synthesis of materials: MBE, MOCVD, PLM u Charge transport and doping u Evaluate photo cathode (p-type semiconductor surface) vs. photo anode (n-type semiconductor surface) designs u Measurements of band offsets u Fundamental studies in-situ and ex-situ of the electrolyte-semiconductor interface u Surface modification u Kinetic H 2 production and corrosion rates. LBNL solar workshop
Future continuous tense and future perfect exercises
Future simple continuous perfect
Quantum computing: progress and prospects
In paragraph 1 impatient of is best interpreted as meaning
Prospects of agriculture in bangladesh
World population prospects
Prospects preposition
Global semiconductor packaging materials outlook
Semiconductor materials
Semiconductor industry structure
Wholesale solar cell panel
Solar energy is free. solar is inexhaustible
Natural materials
Harmful or useful
Man made materials
What is adopting materials
Energy future lines solar panelsstoragebatteries
The future of solar energy
See future continuous
Tenses in english
Future plans and finished future actions
Future perfect x
Nulti kondicional
Direct materials budget with multiple materials
Future perfect presentation
Simple future work
Future nurse future midwife e learning
Present continuous future
Present continuous for future plan