Ch 18 Ethers and Epoxides Thiols and Sulfides
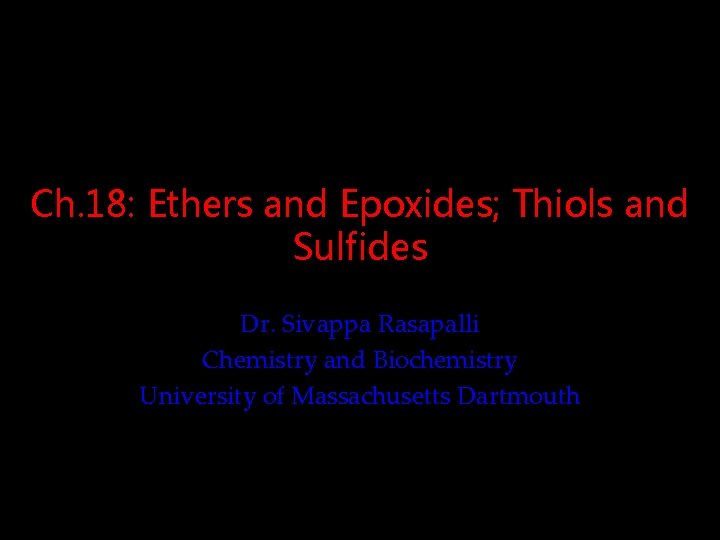
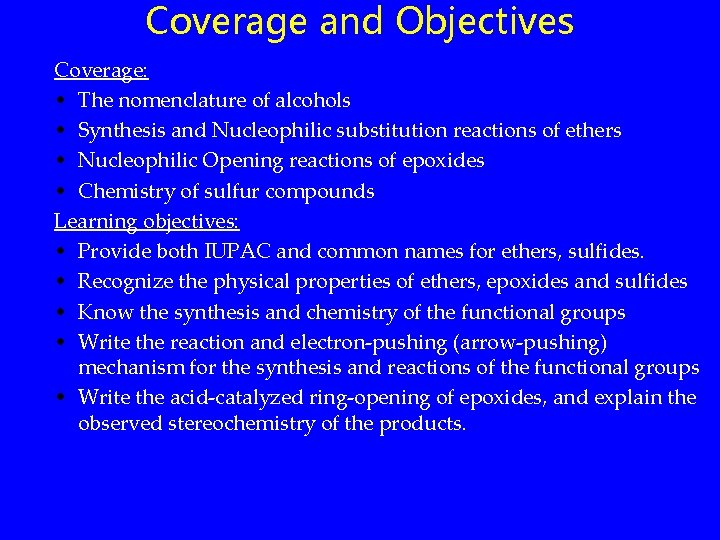
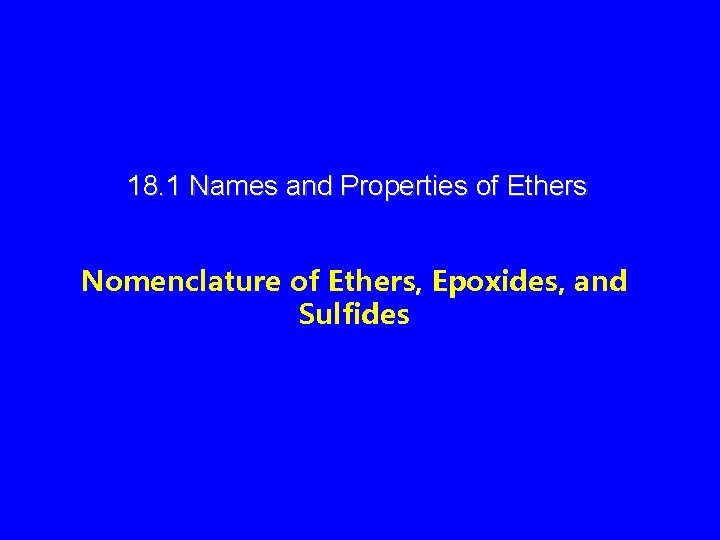
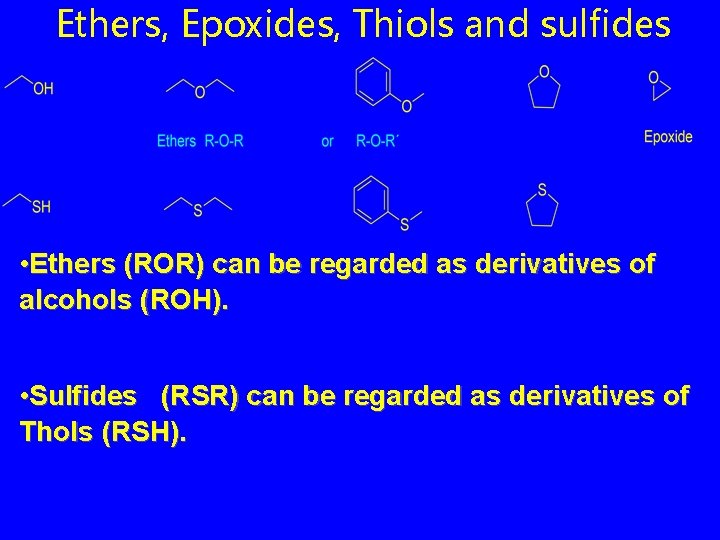
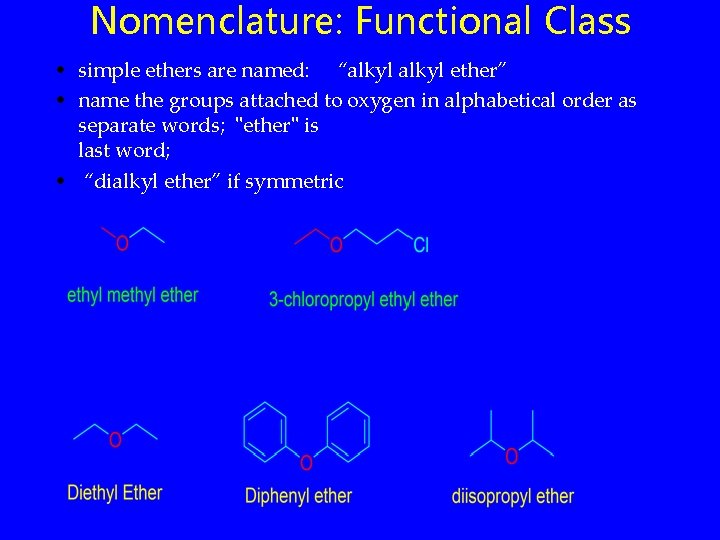
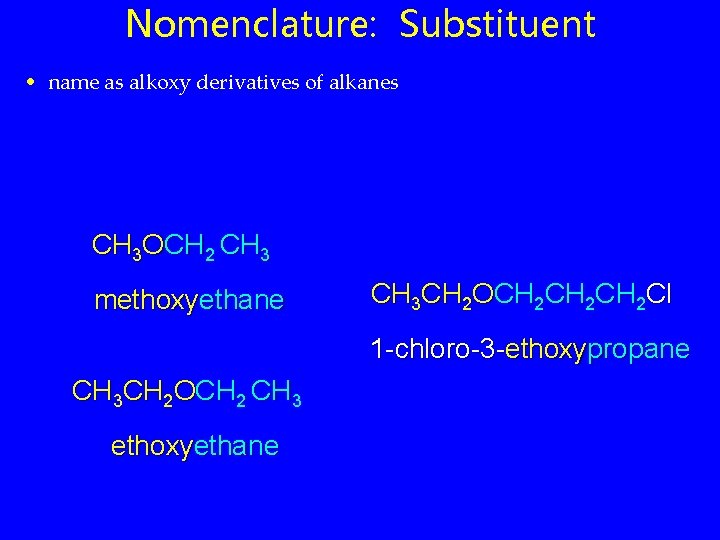
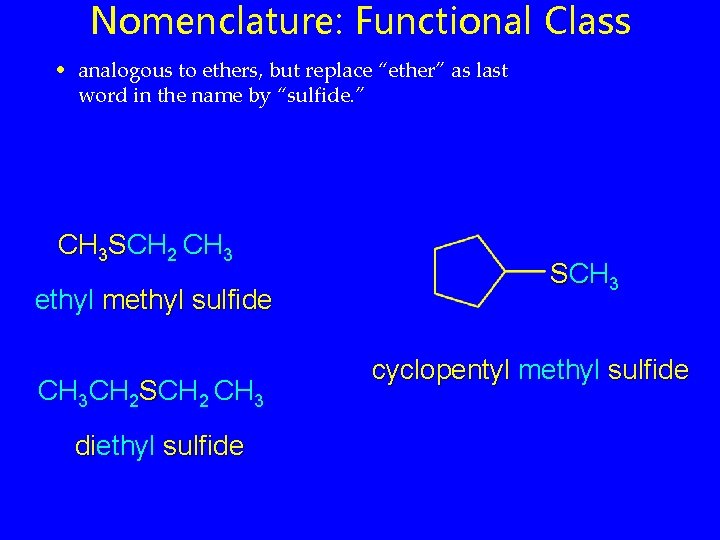
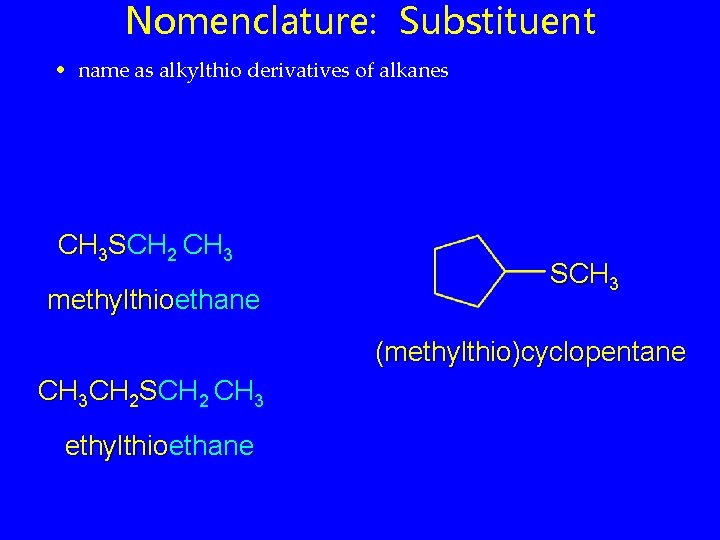
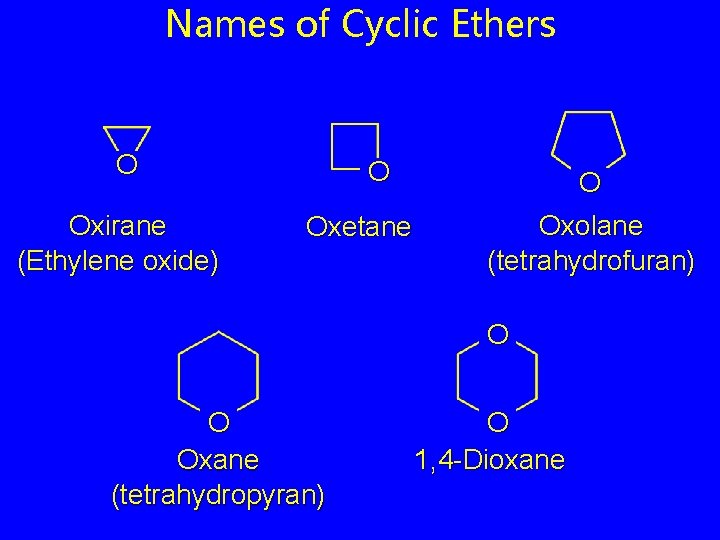
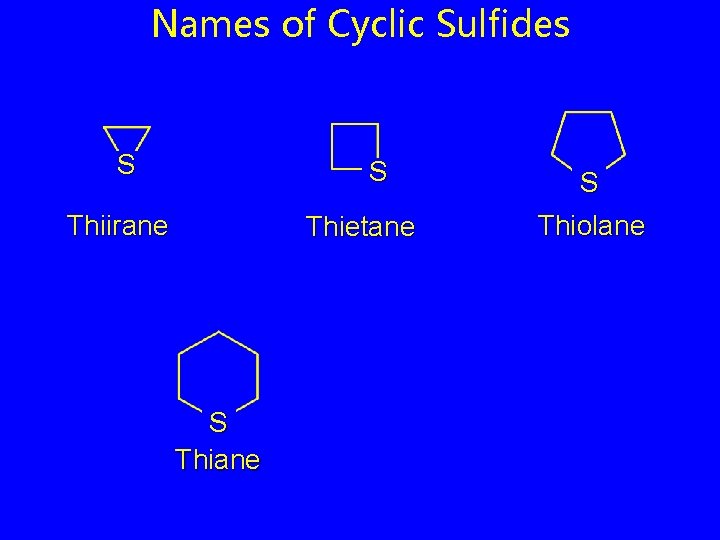
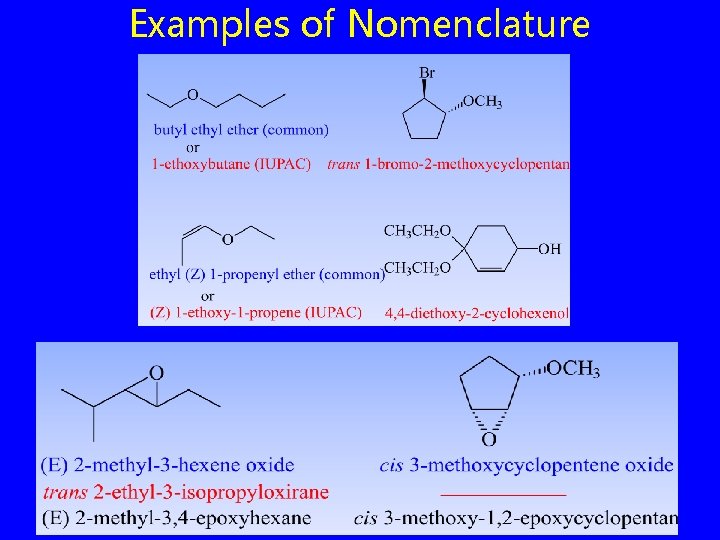
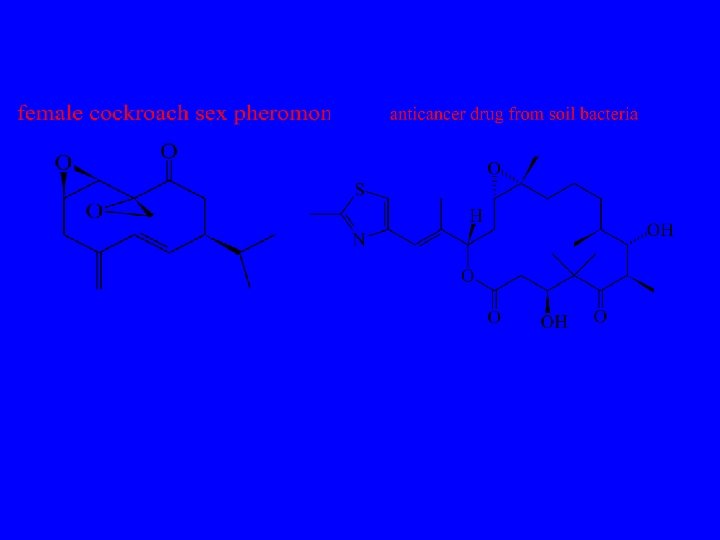
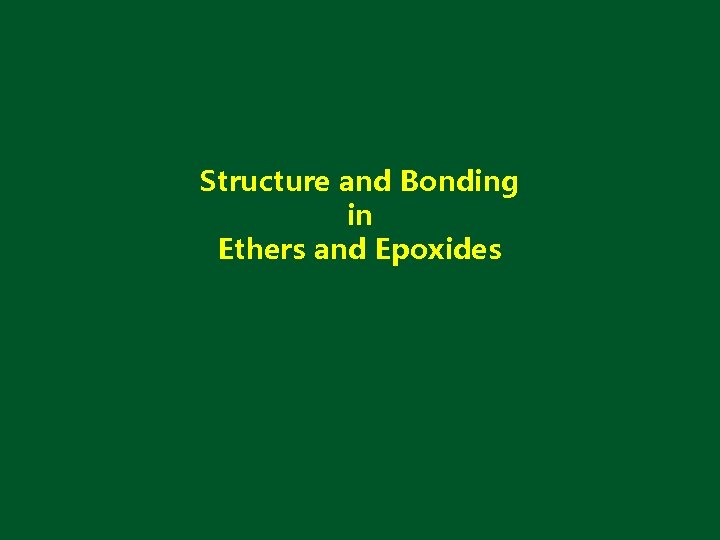
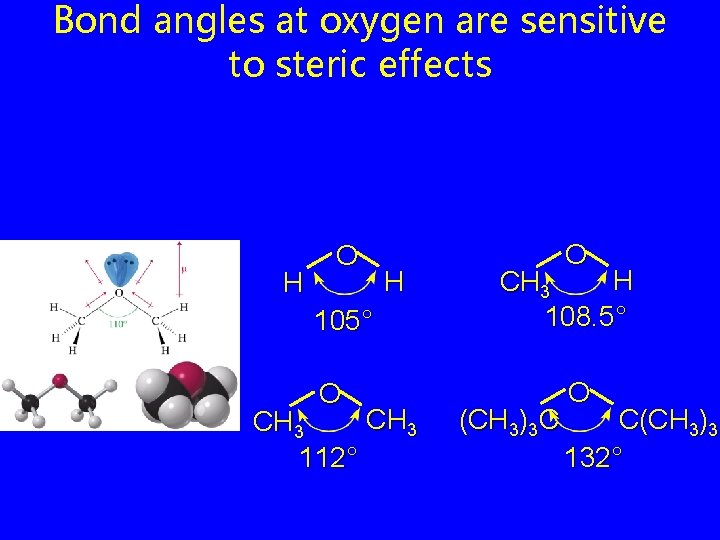
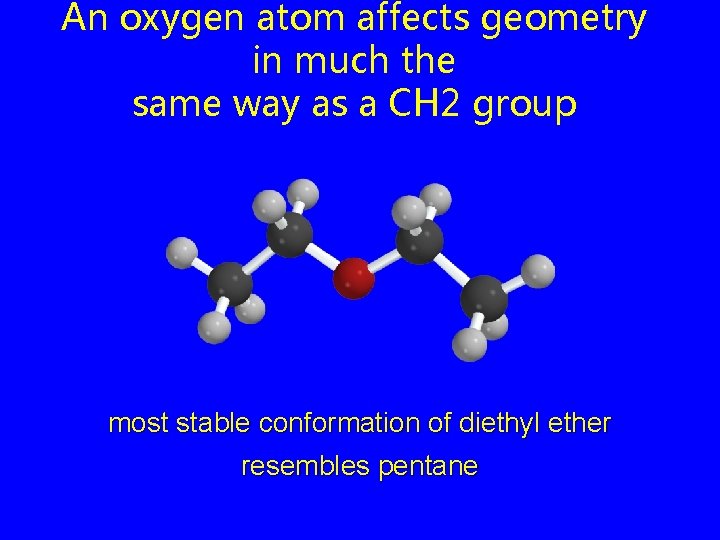
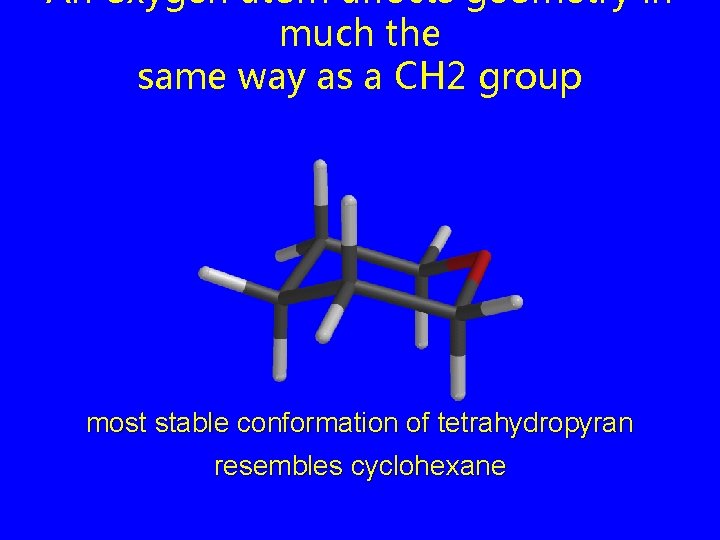
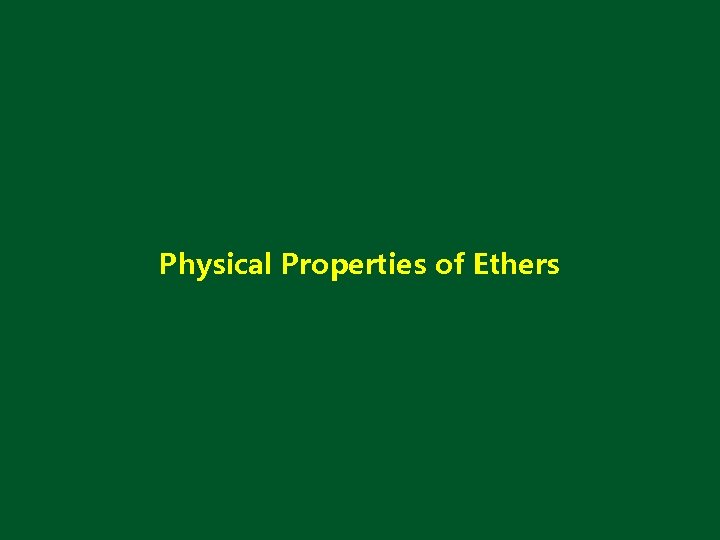
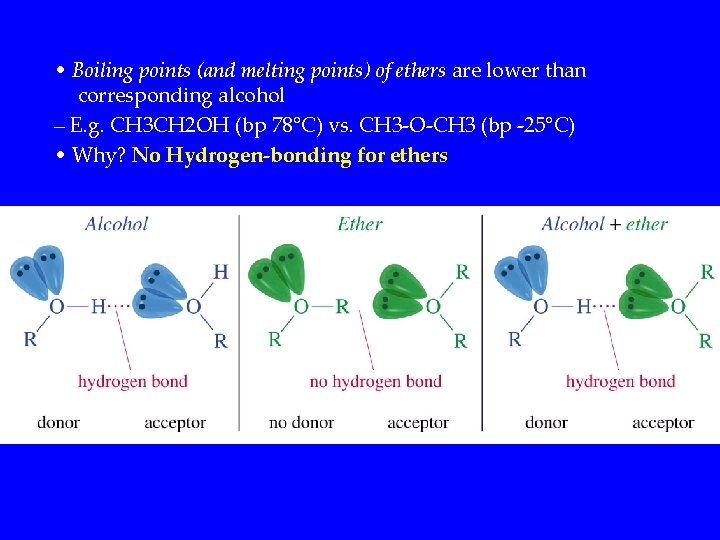
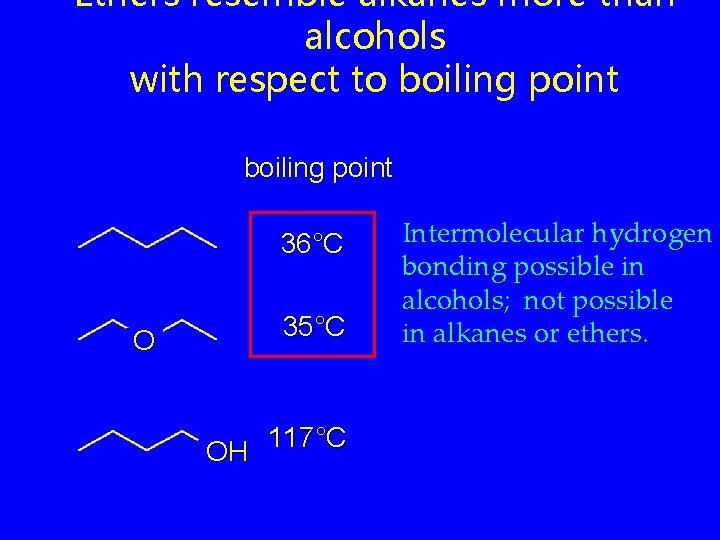
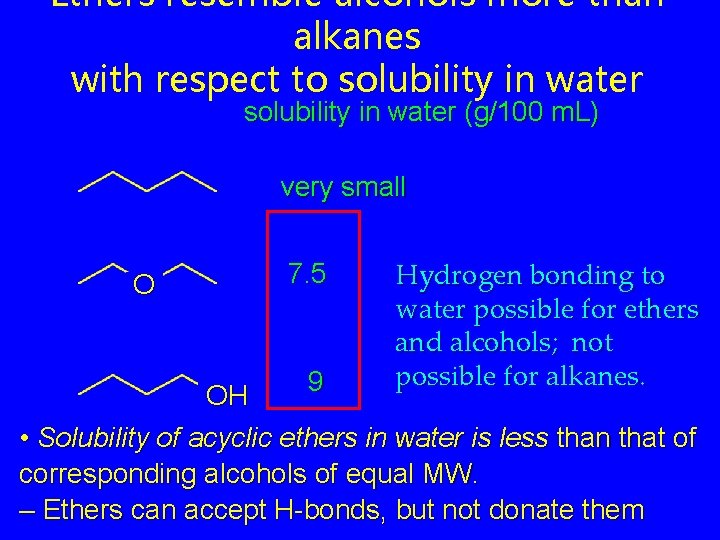
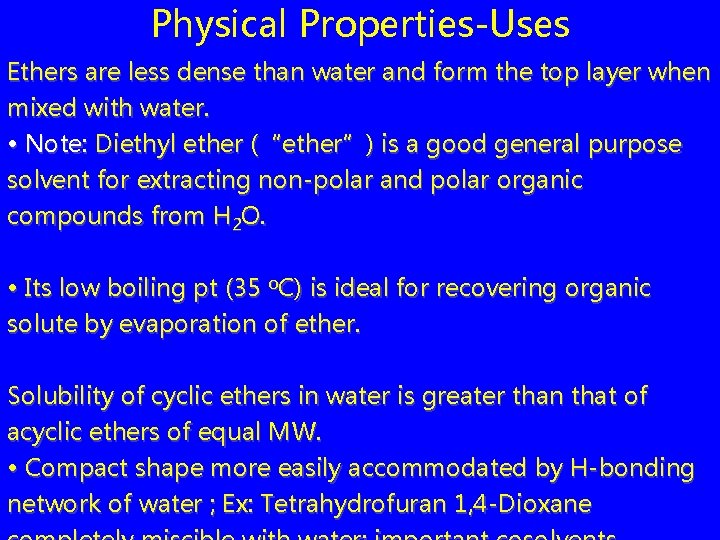
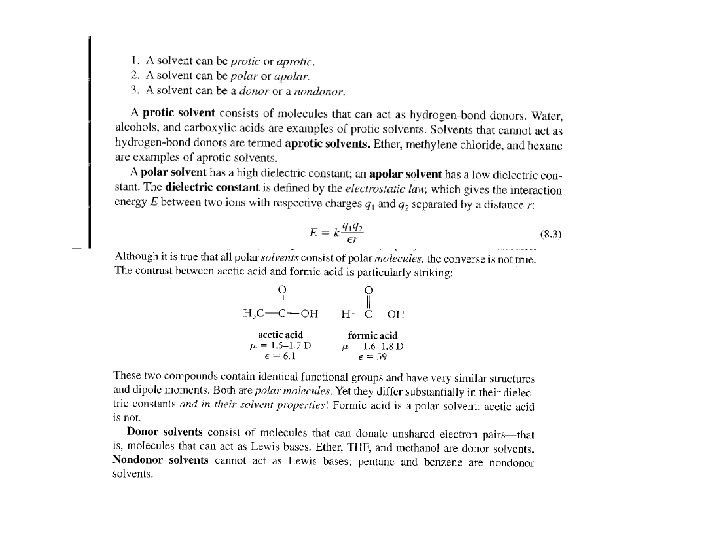
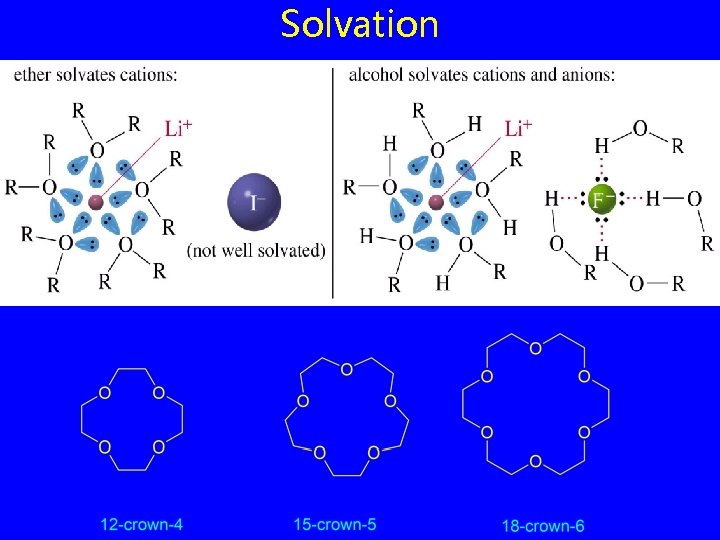
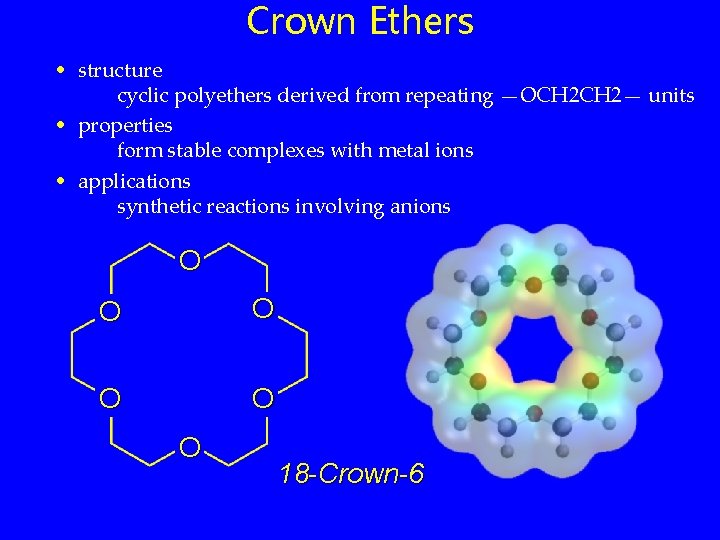
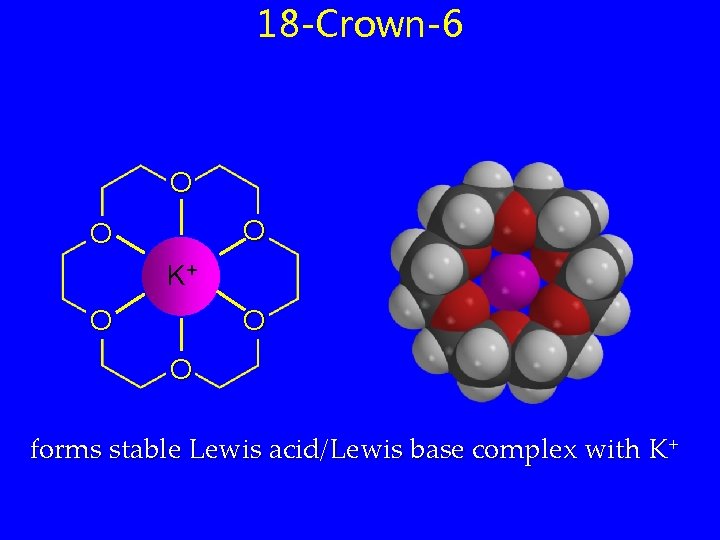
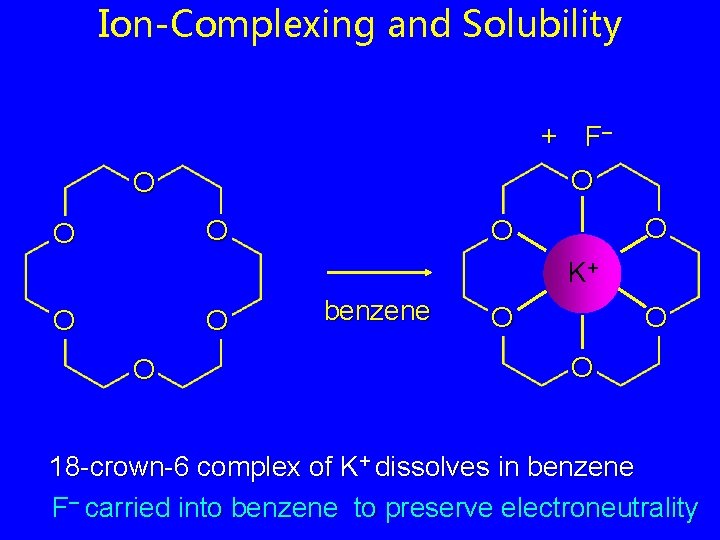
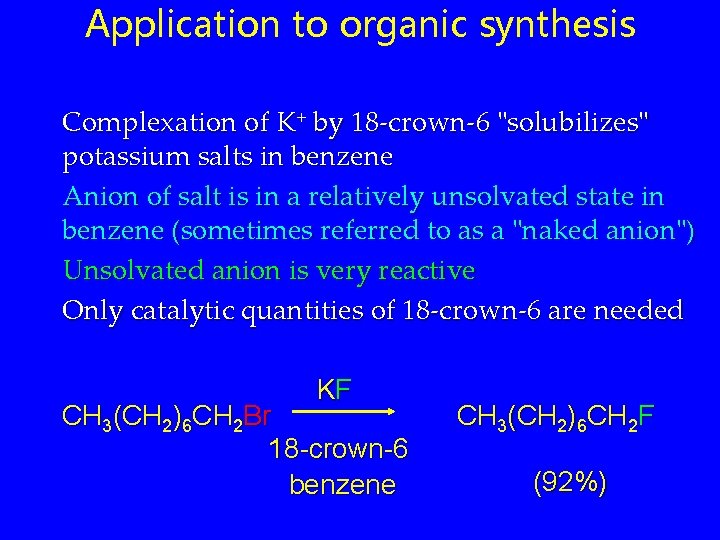
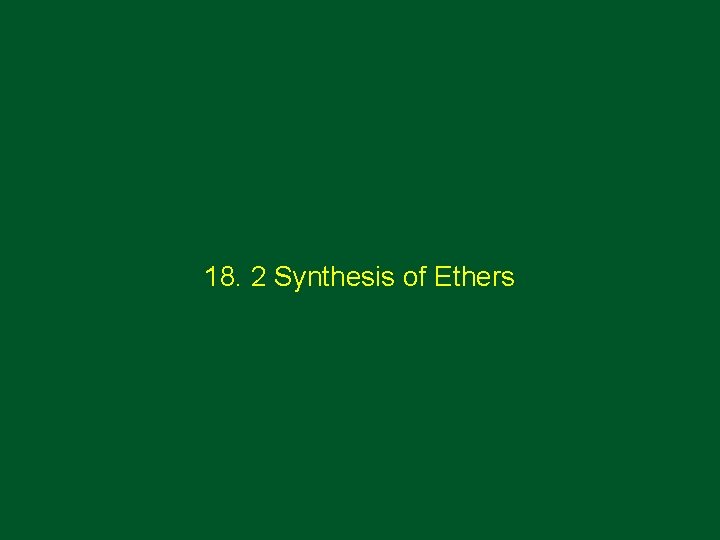
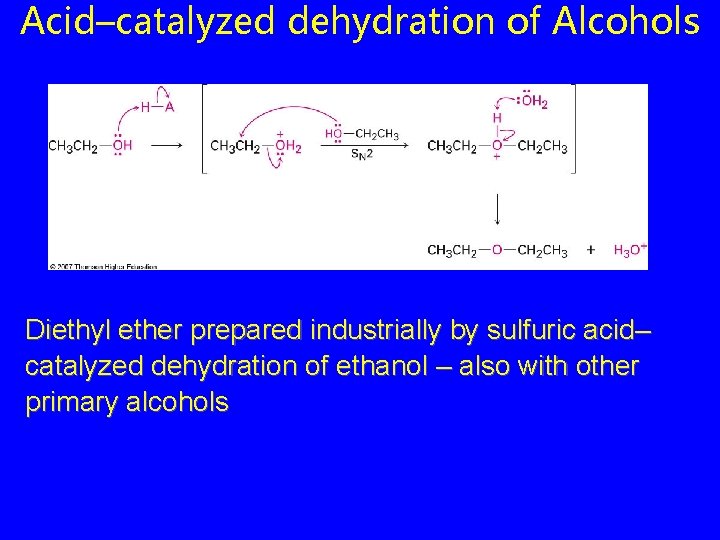
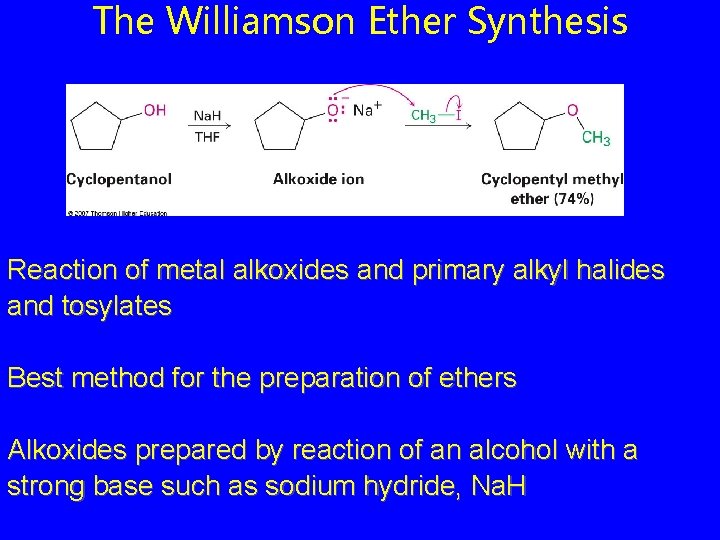
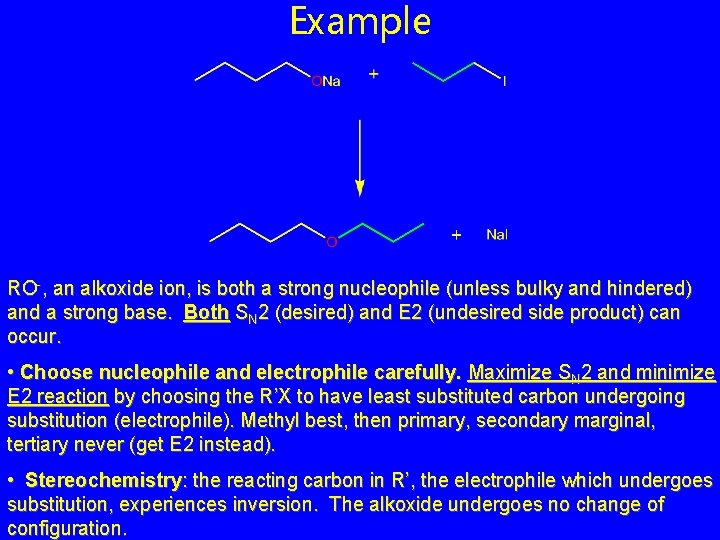
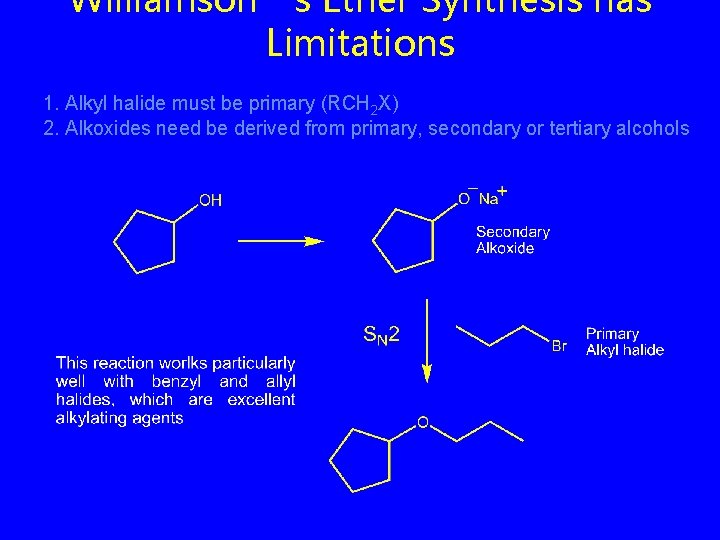
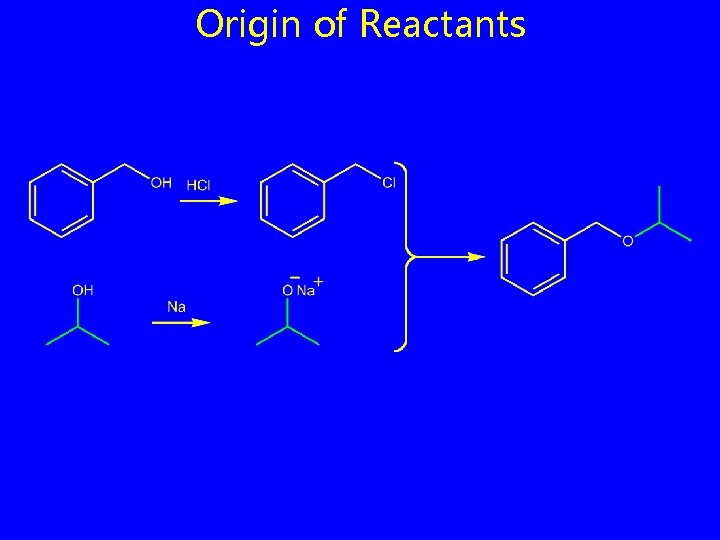
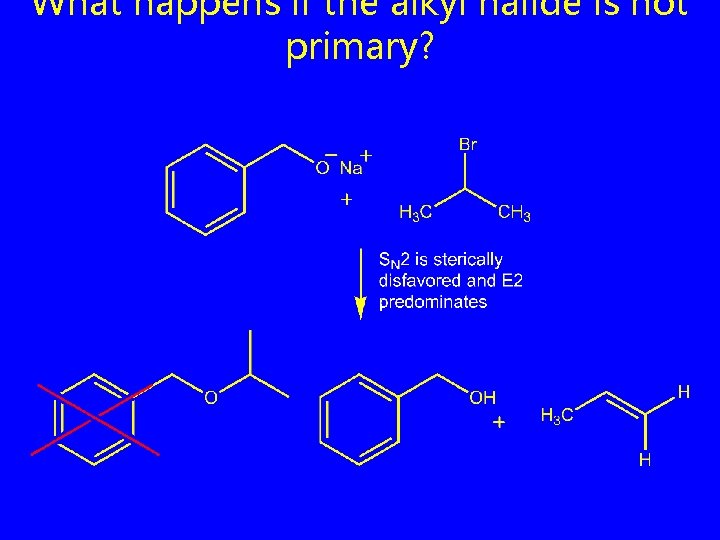
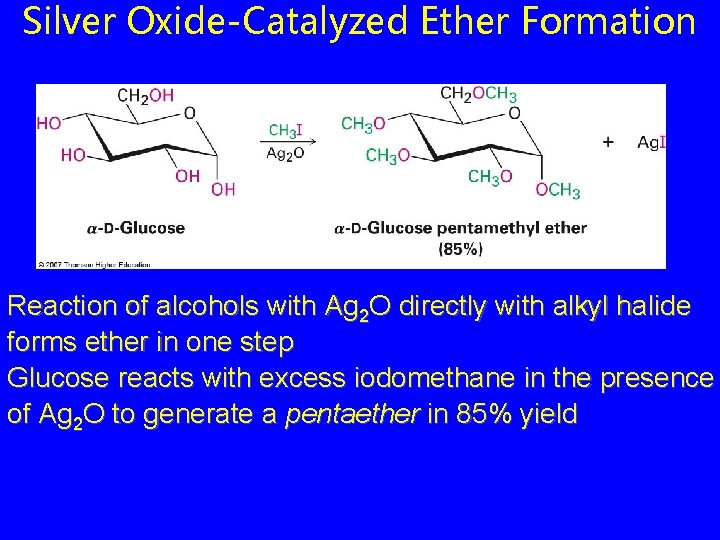
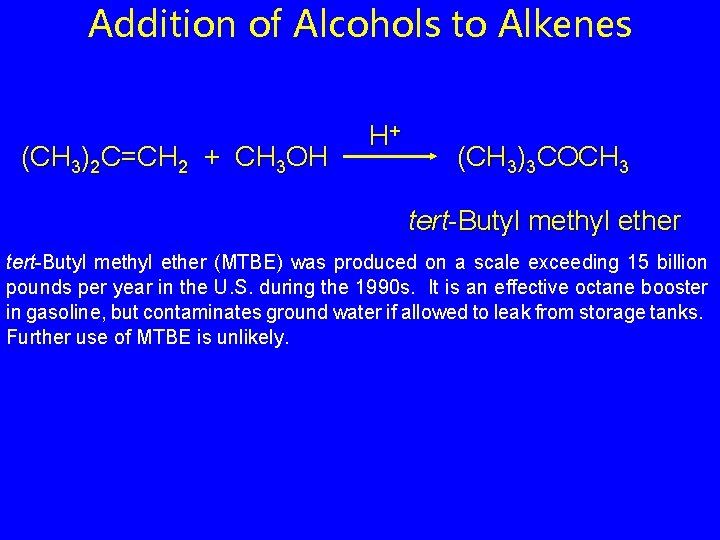
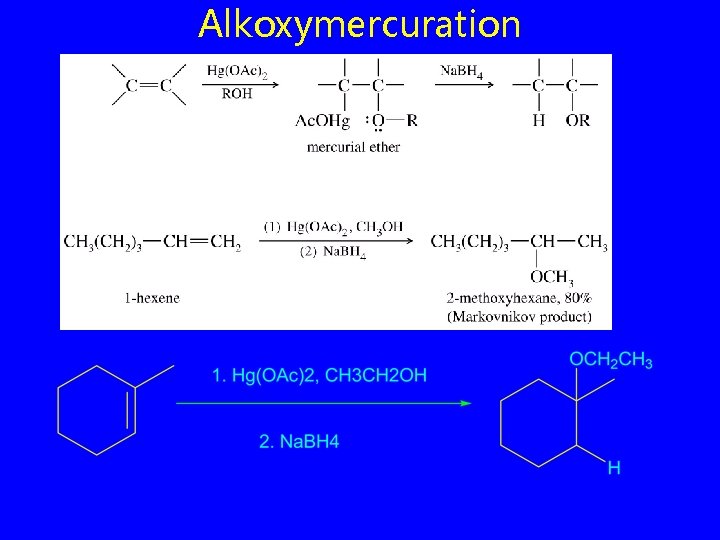
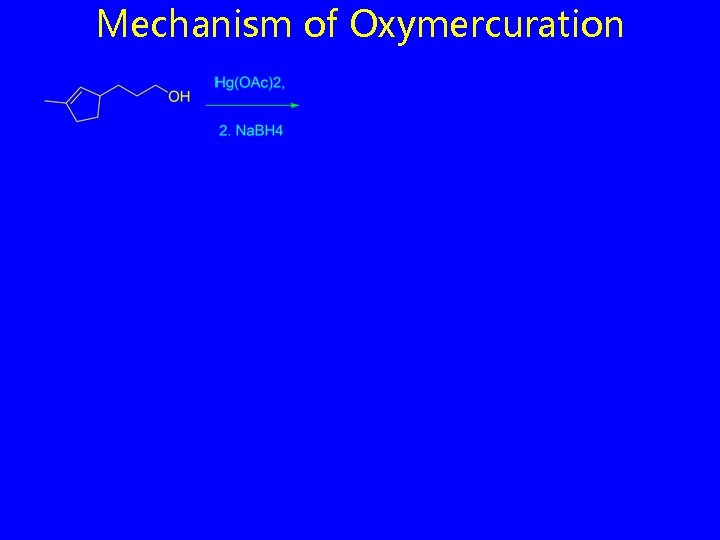
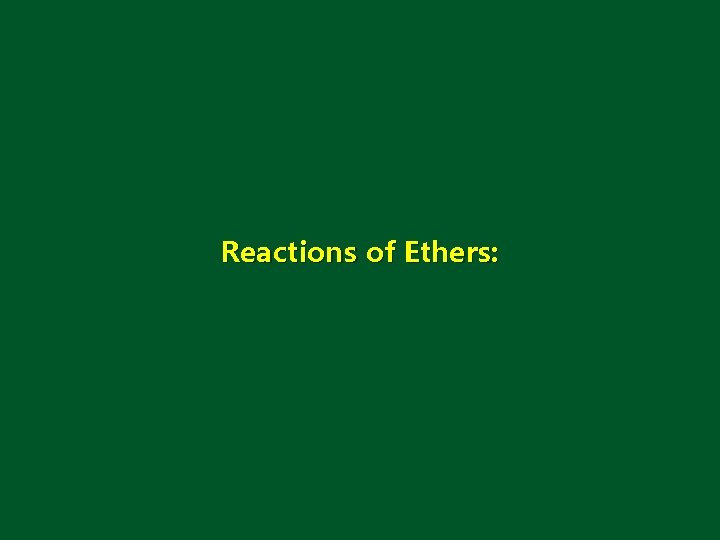
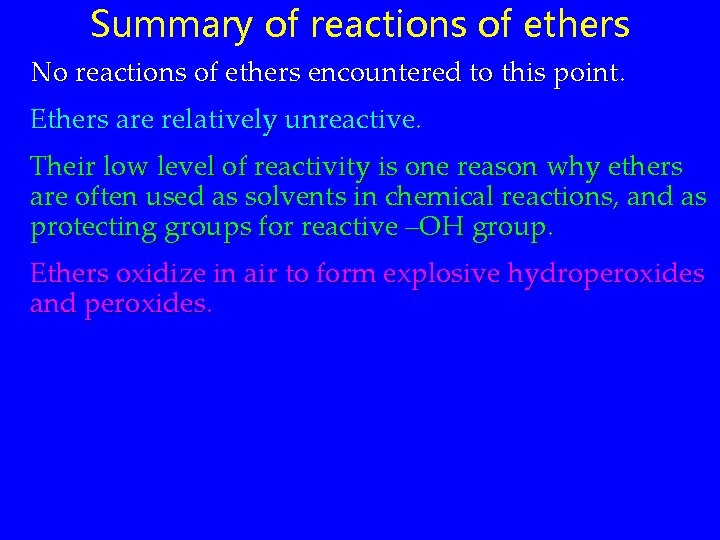
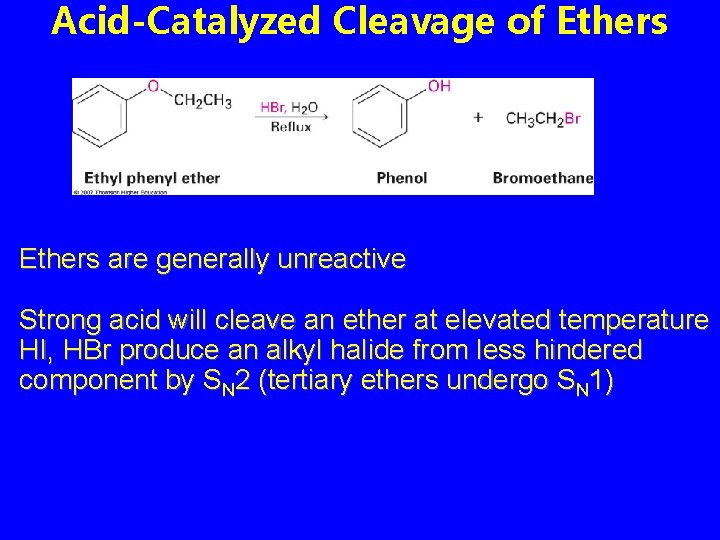
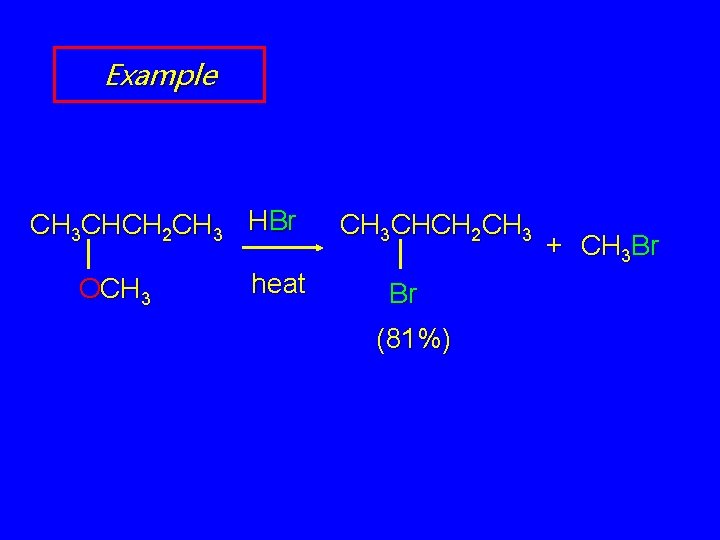
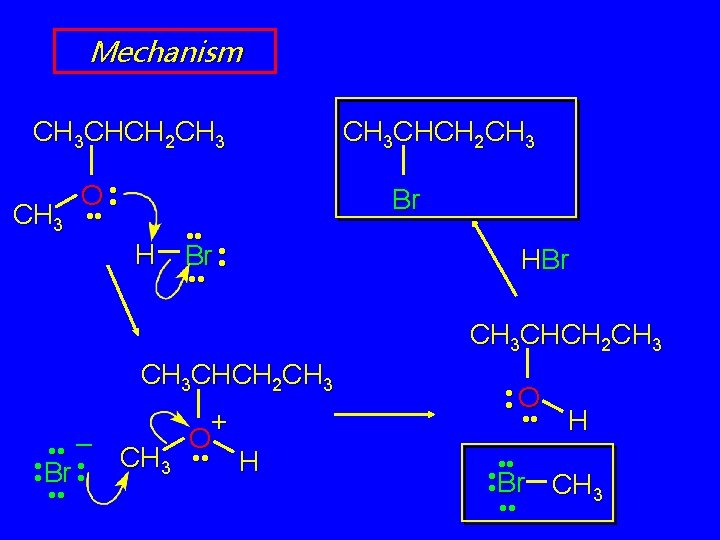
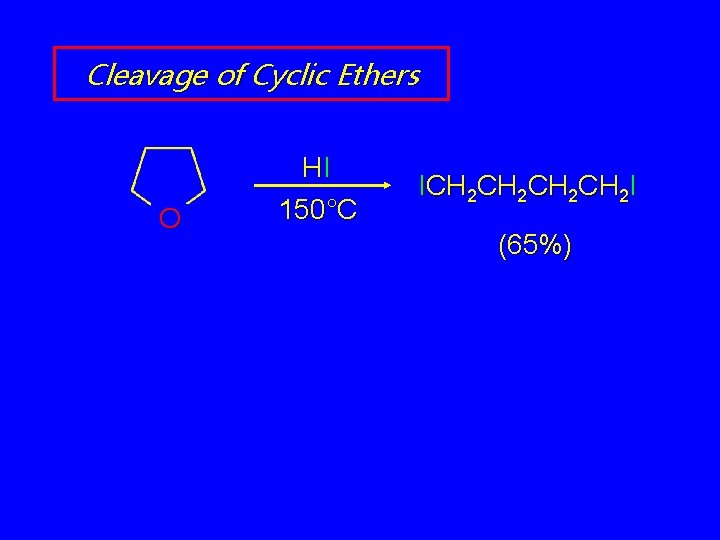
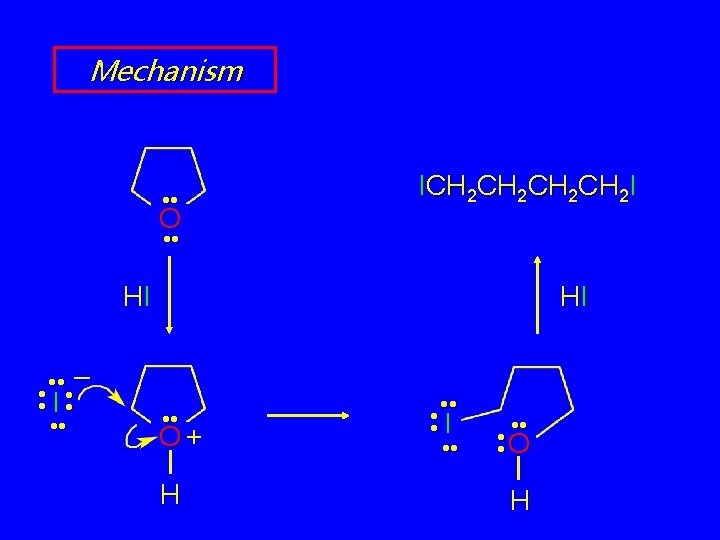
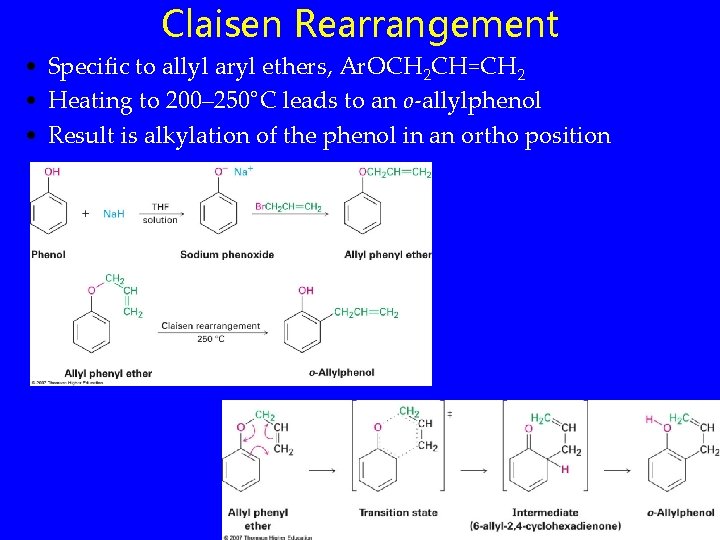
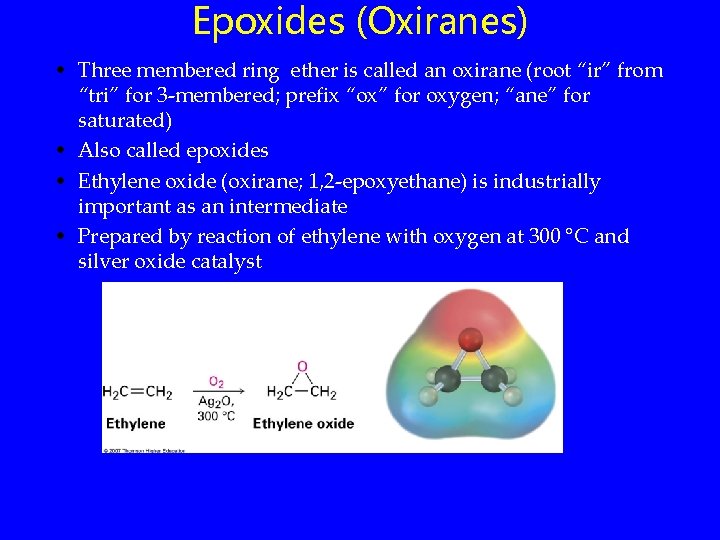
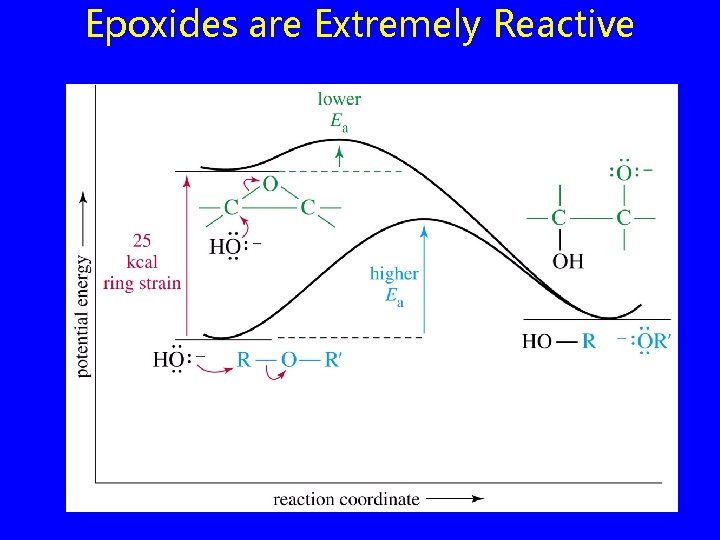
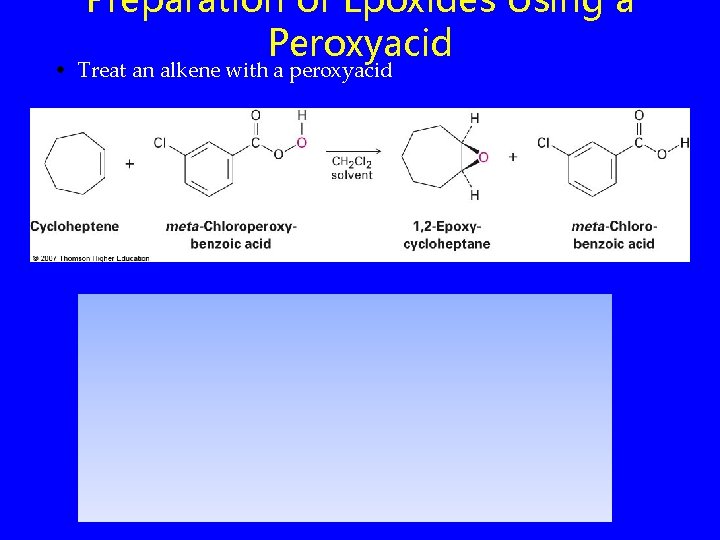
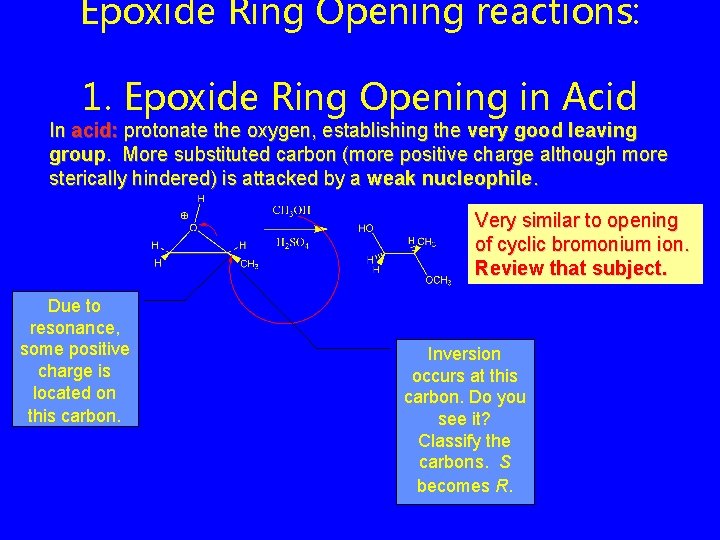
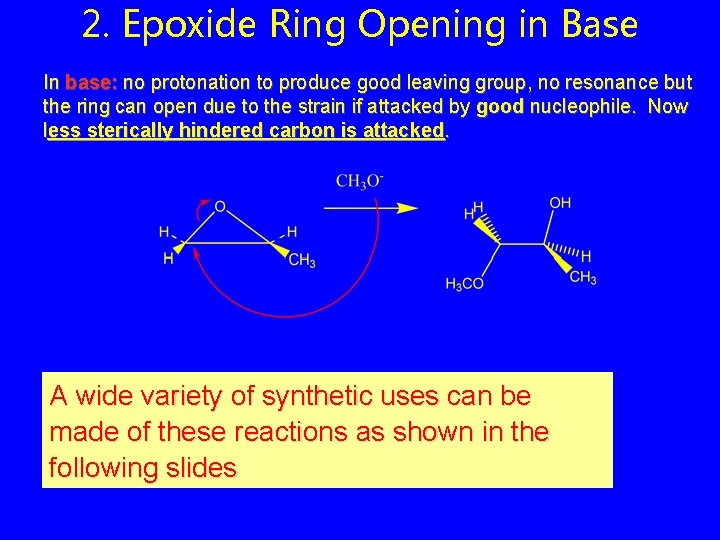
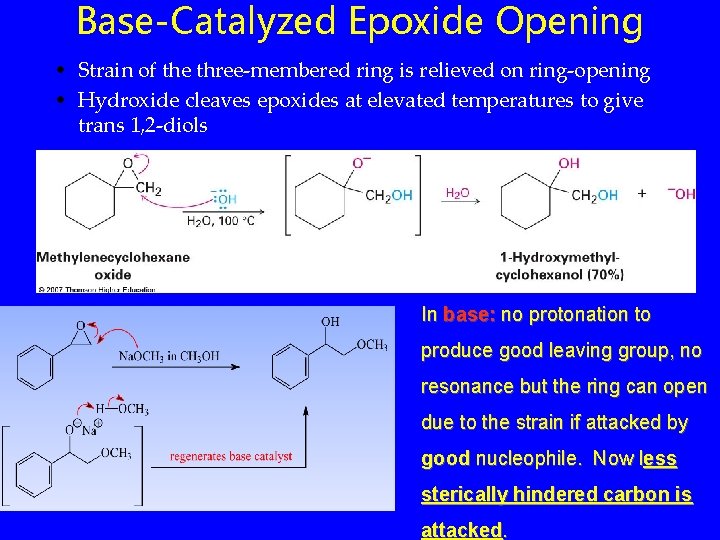
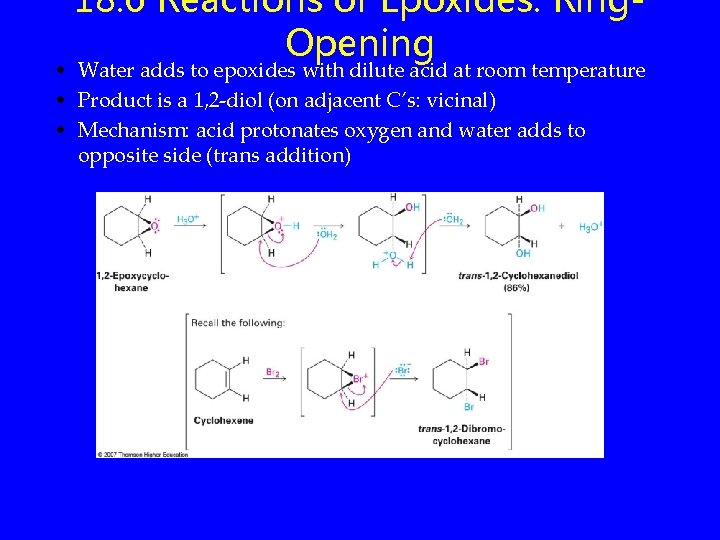
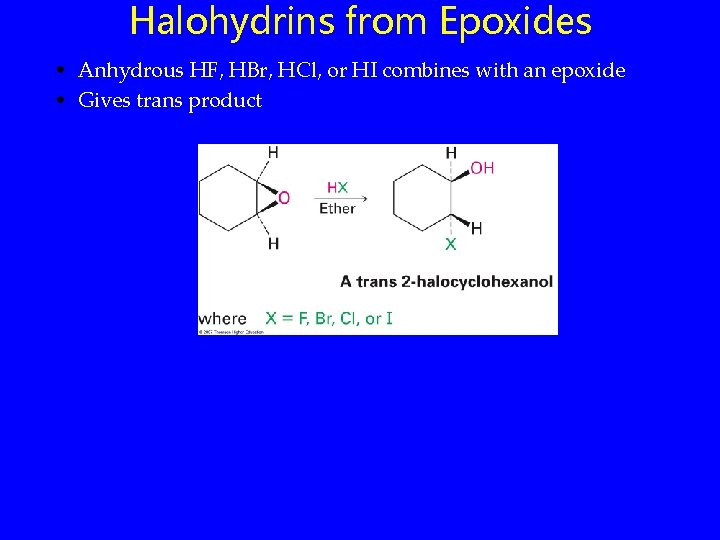
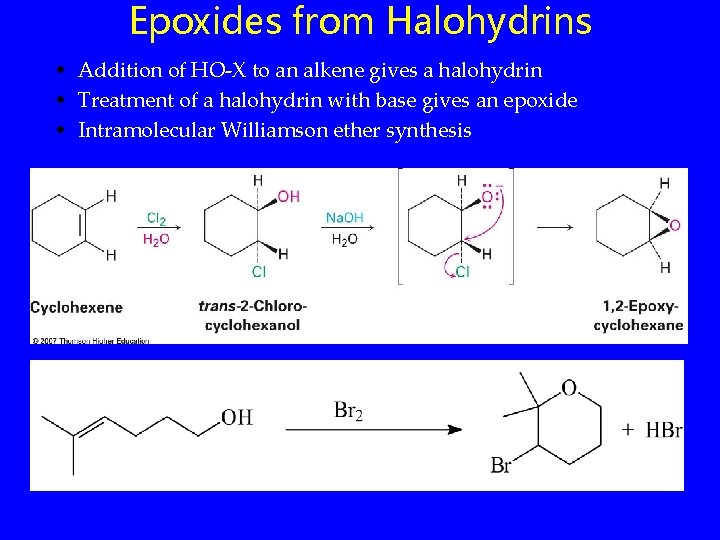
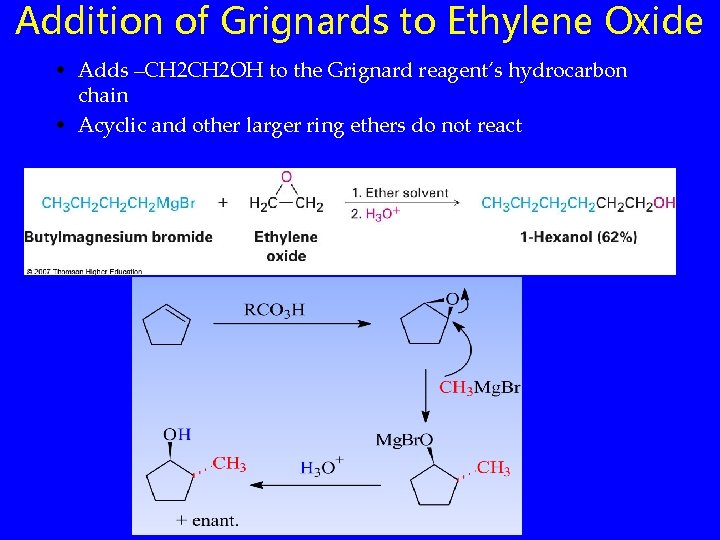
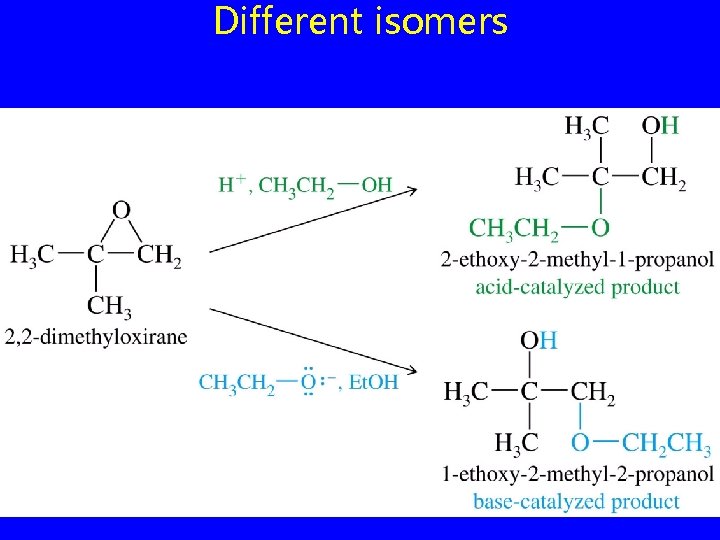
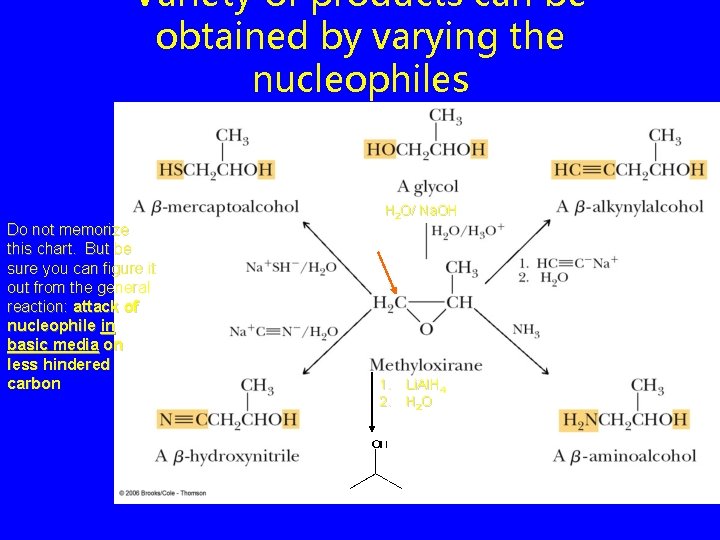
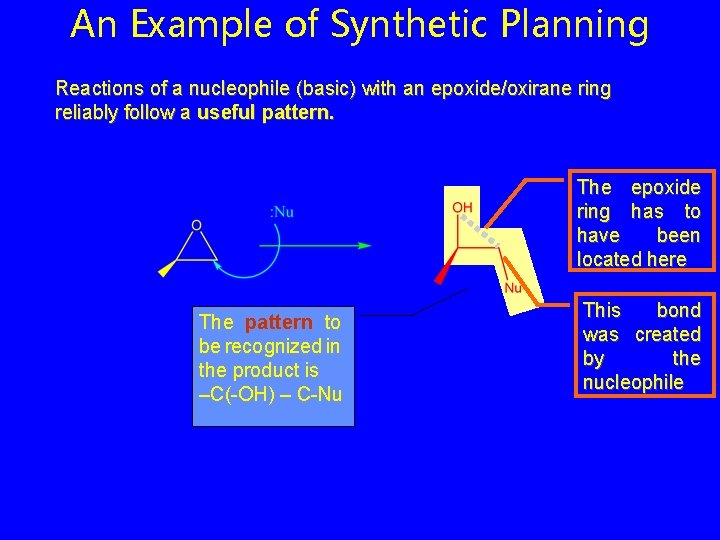
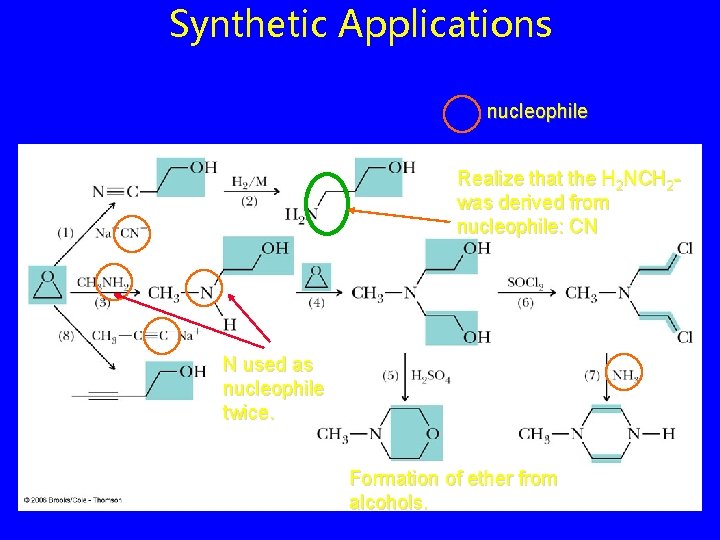
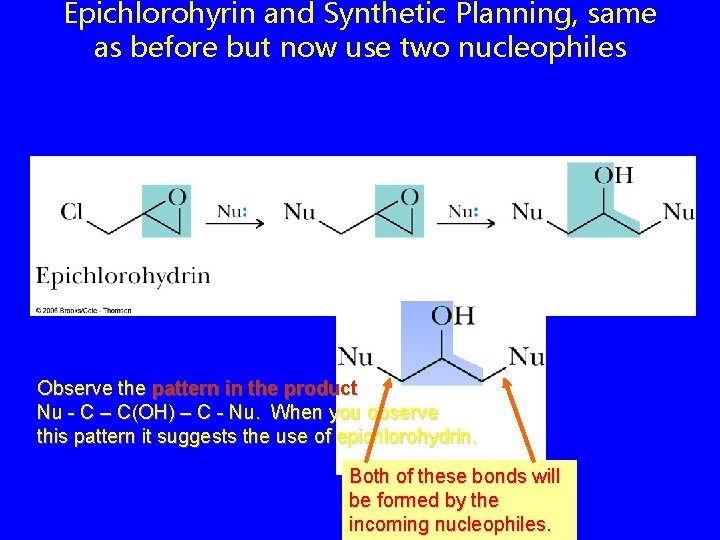
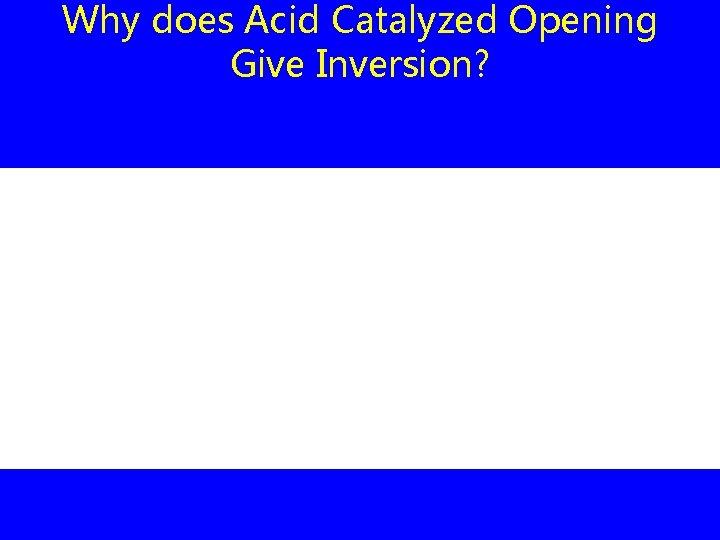
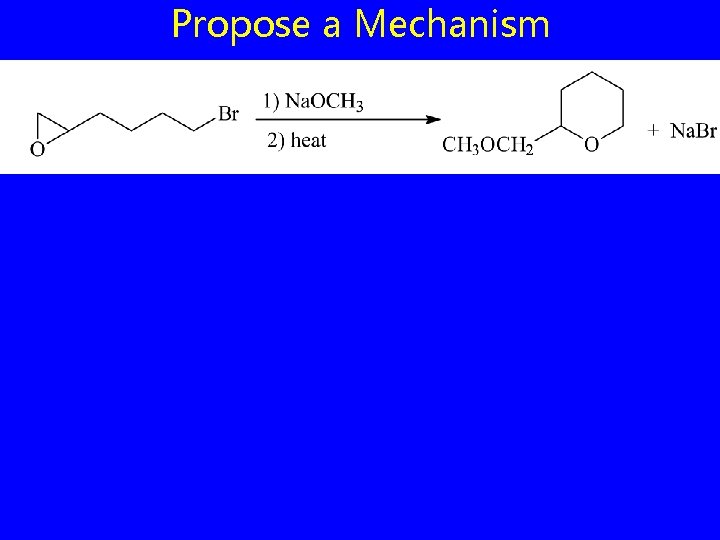
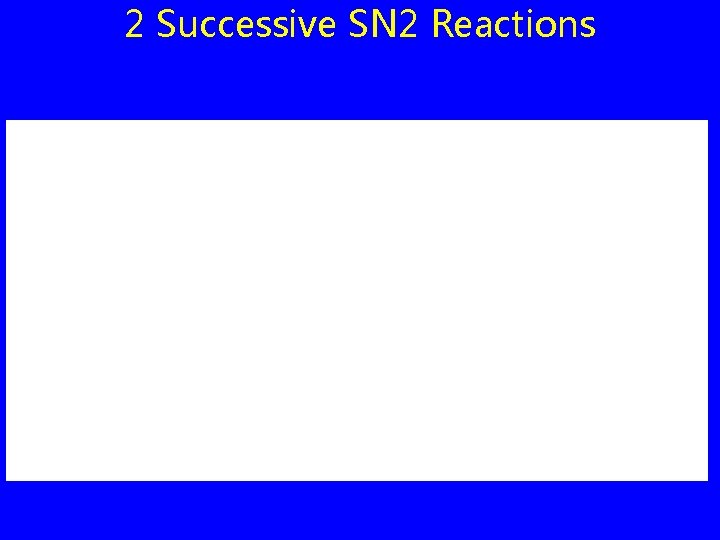
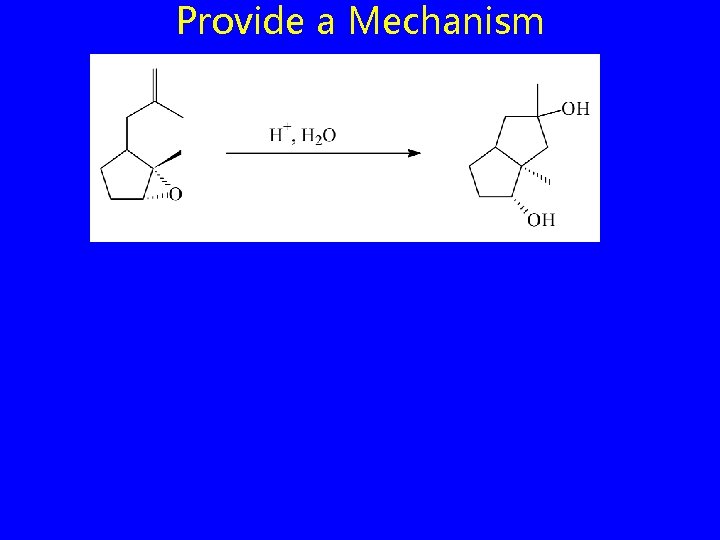
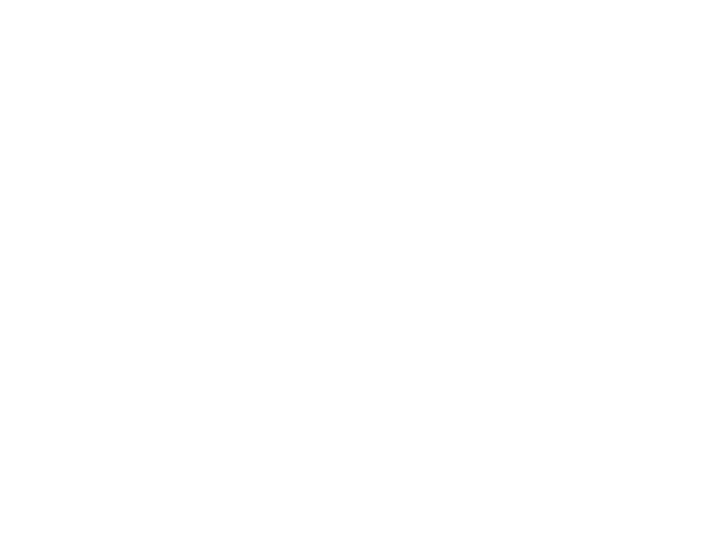
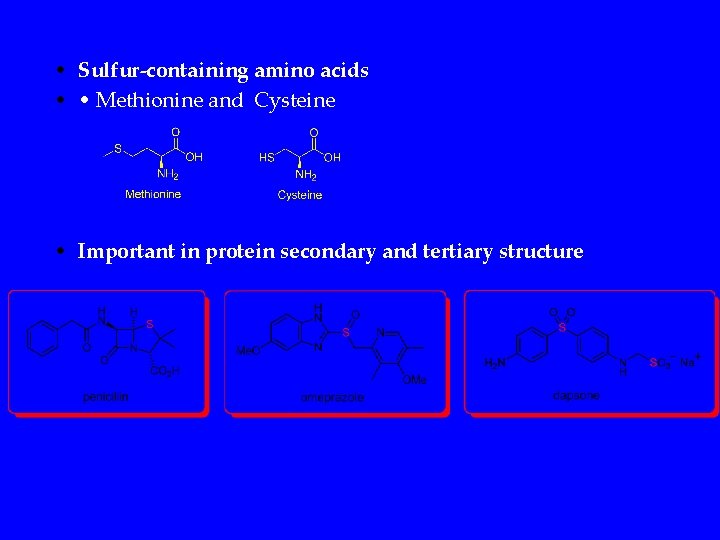
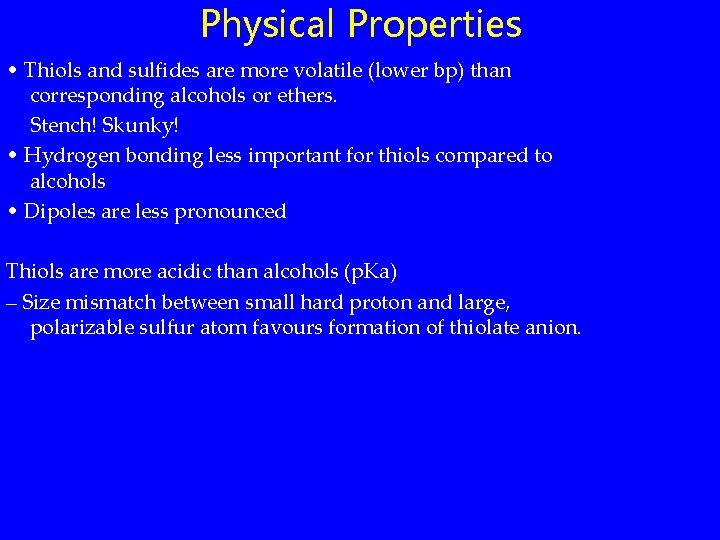
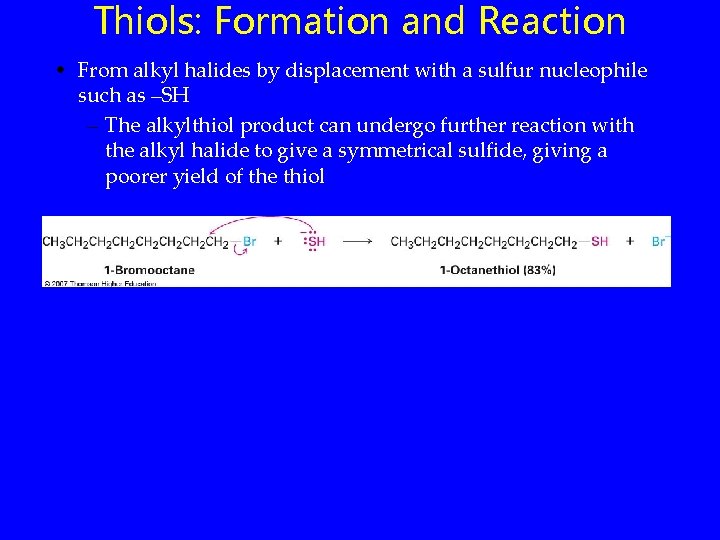
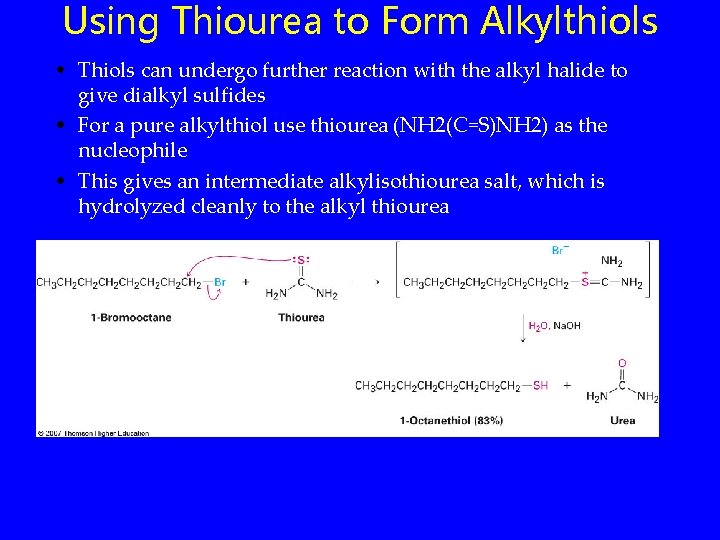
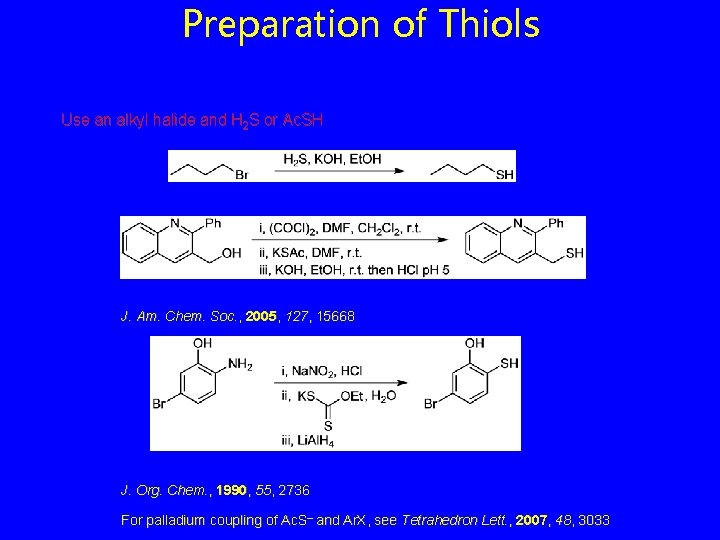
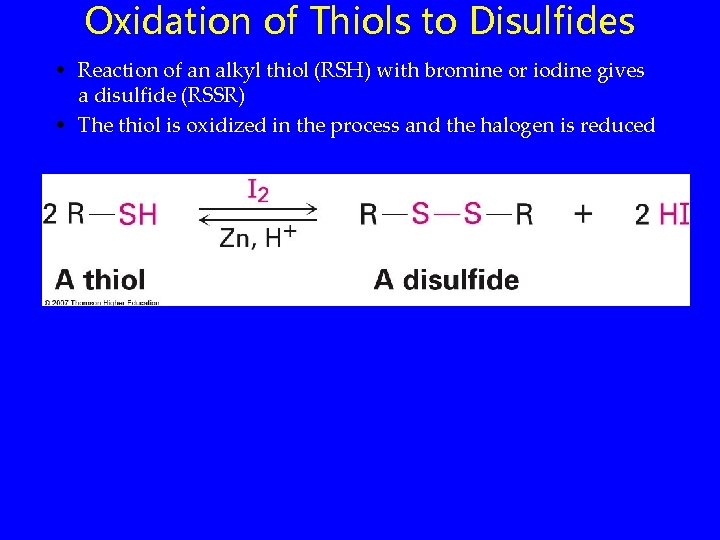
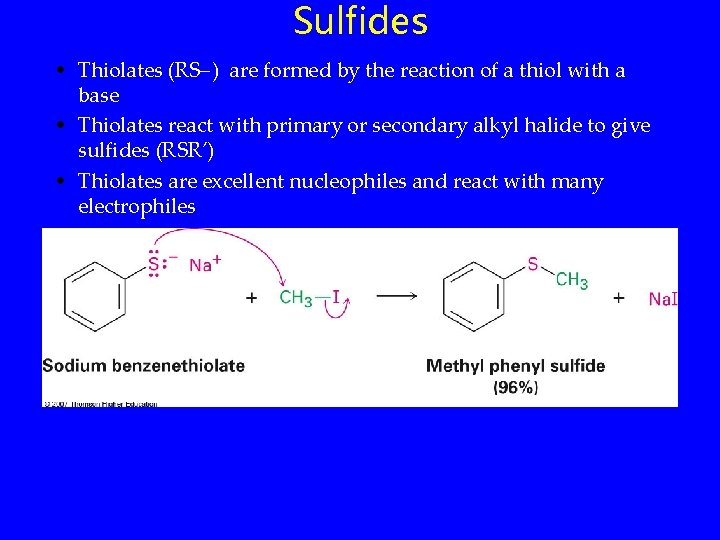
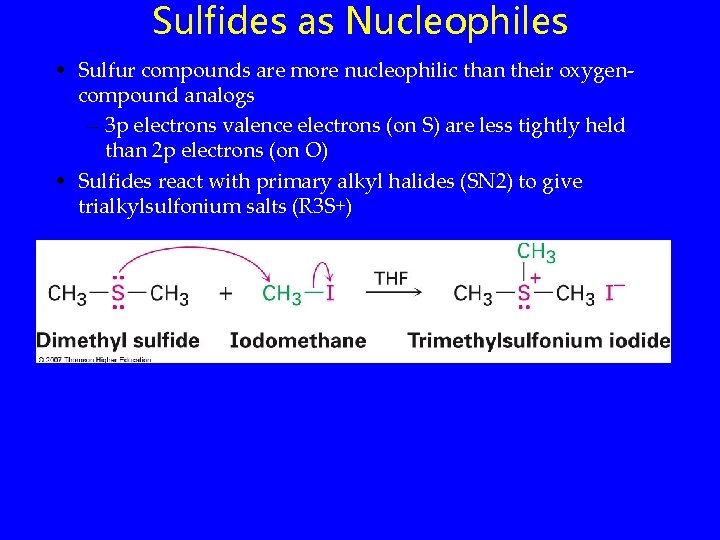
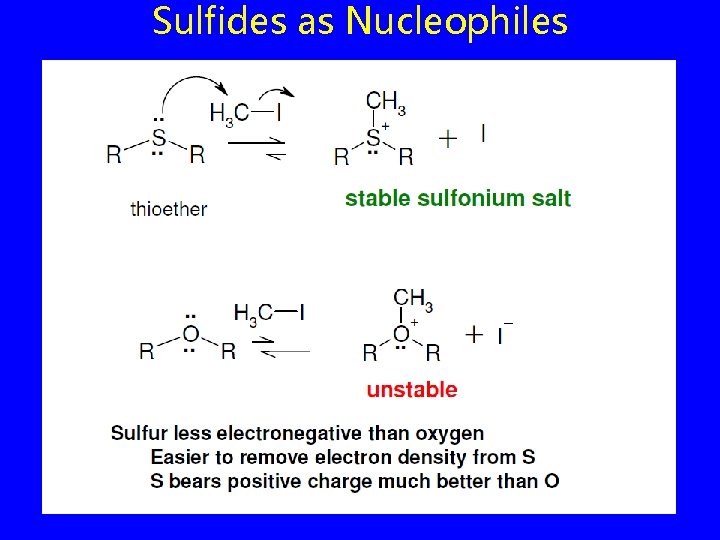
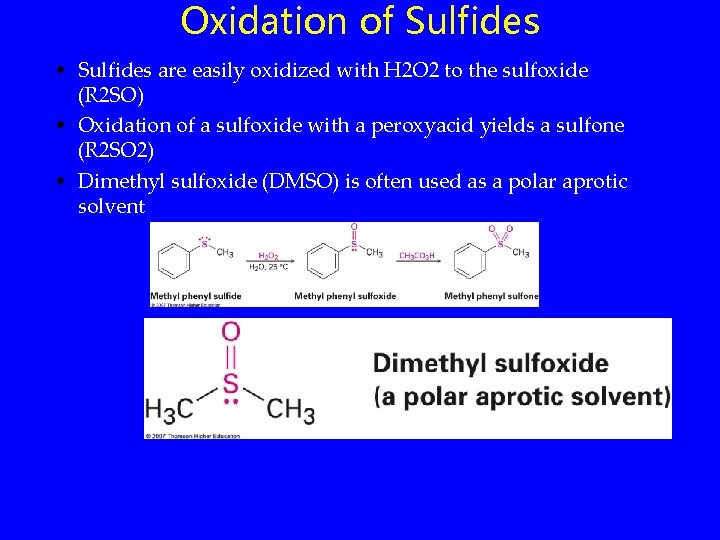
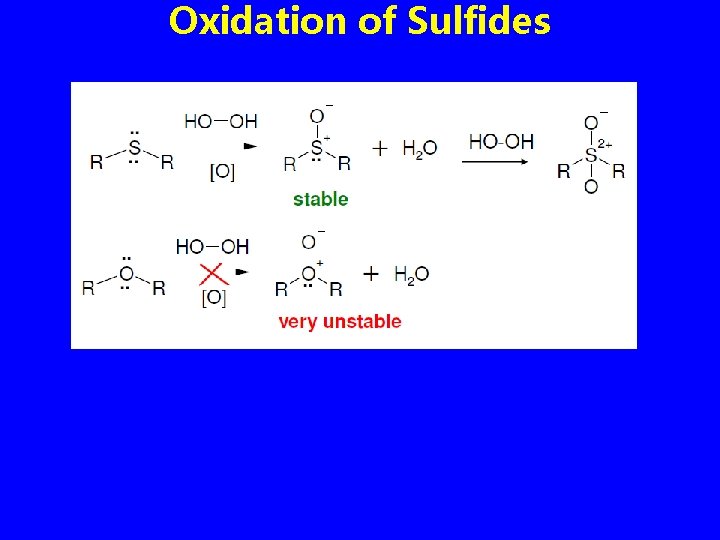
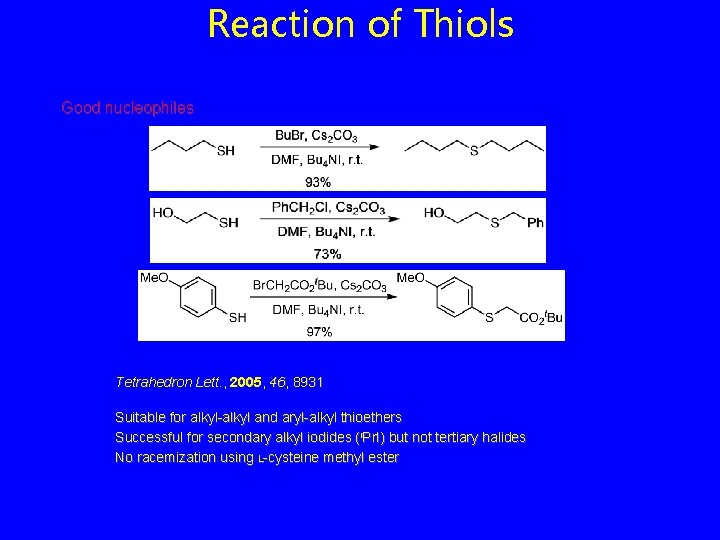
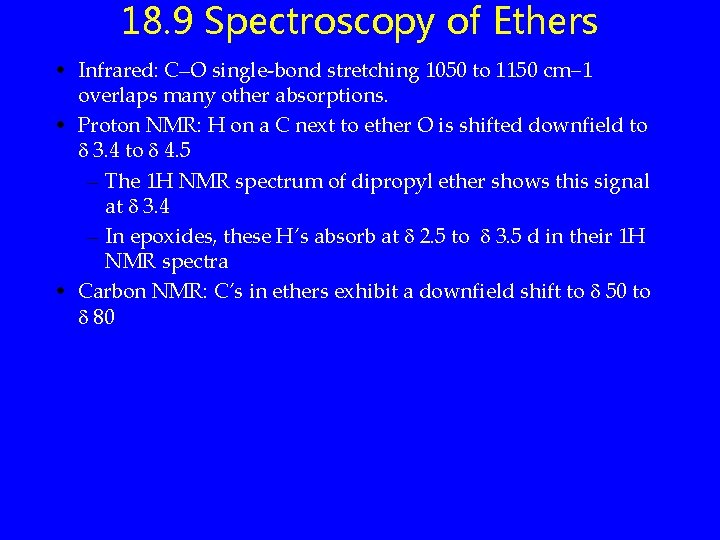
- Slides: 79
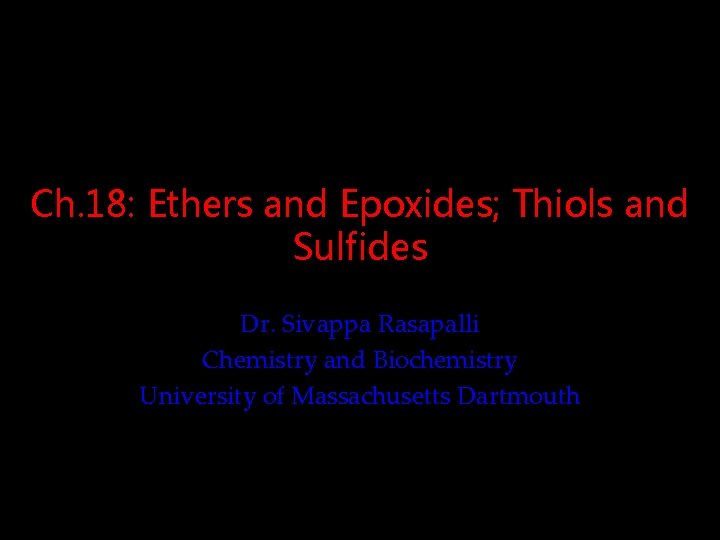
Ch. 18: Ethers and Epoxides; Thiols and Sulfides Dr. Sivappa Rasapalli Chemistry and Biochemistry University of Massachusetts Dartmouth
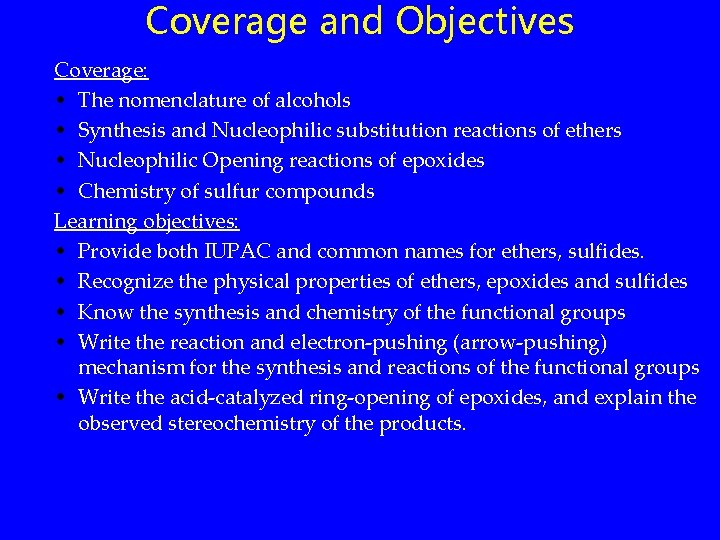
Coverage and Objectives Coverage: • The nomenclature of alcohols • Synthesis and Nucleophilic substitution reactions of ethers • Nucleophilic Opening reactions of epoxides • Chemistry of sulfur compounds Learning objectives: • Provide both IUPAC and common names for ethers, sulfides. • Recognize the physical properties of ethers, epoxides and sulfides • Know the synthesis and chemistry of the functional groups • Write the reaction and electron-pushing (arrow-pushing) mechanism for the synthesis and reactions of the functional groups • Write the acid-catalyzed ring-opening of epoxides, and explain the observed stereochemistry of the products.
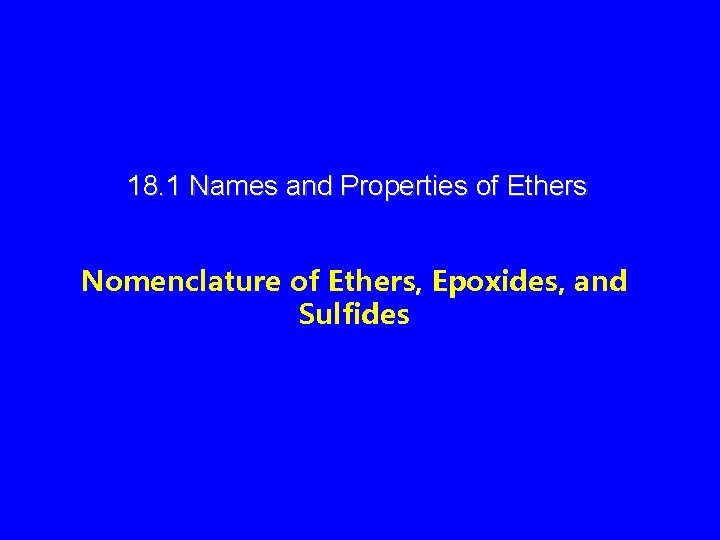
18. 1 Names and Properties of Ethers Nomenclature of Ethers, Epoxides, and Sulfides
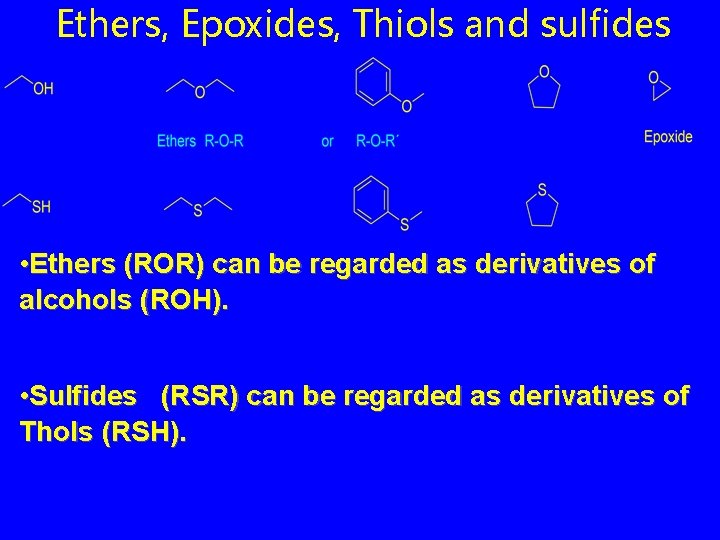
Ethers, Epoxides, Thiols and sulfides • Ethers (ROR) can be regarded as derivatives of alcohols (ROH). • Sulfides (RSR) can be regarded as derivatives of Thols (RSH).
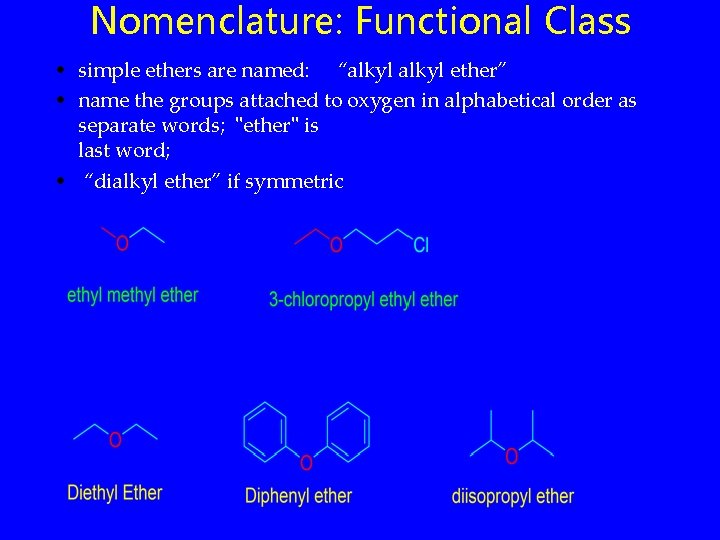
Nomenclature: Functional Class • simple ethers are named: “alkyl ether” • name the groups attached to oxygen in alphabetical order as separate words; "ether" is last word; • “dialkyl ether” if symmetric
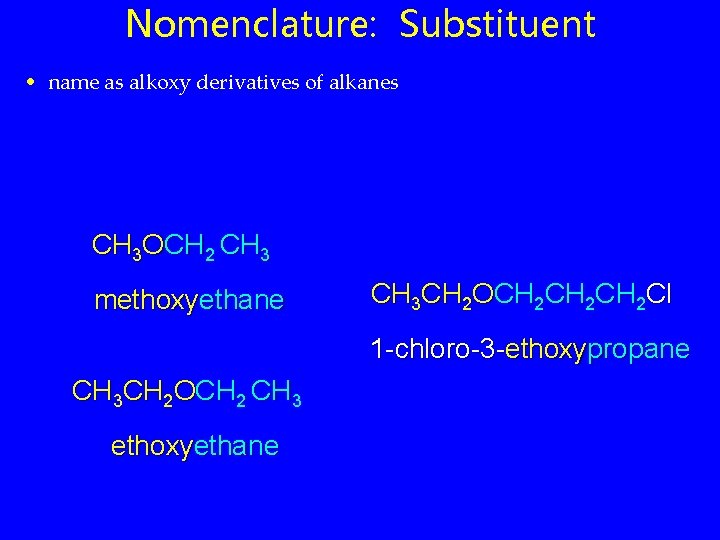
Nomenclature: Substituent • name as alkoxy derivatives of alkanes CH 3 OCH 2 CH 3 methoxyethane CH 3 CH 2 OCH 2 CH 2 Cl 1 -chloro-3 -ethoxypropane CH 3 CH 2 OCH 2 CH 3 ethoxyethane
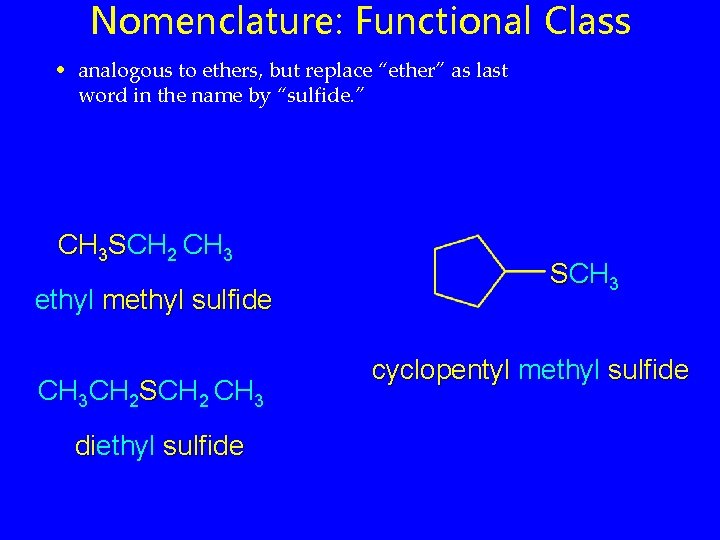
Nomenclature: Functional Class • analogous to ethers, but replace “ether” as last word in the name by “sulfide. ” CH 3 SCH 2 CH 3 ethyl methyl sulfide CH 3 CH 2 SCH 2 CH 3 diethyl sulfide SCH 3 cyclopentyl methyl sulfide
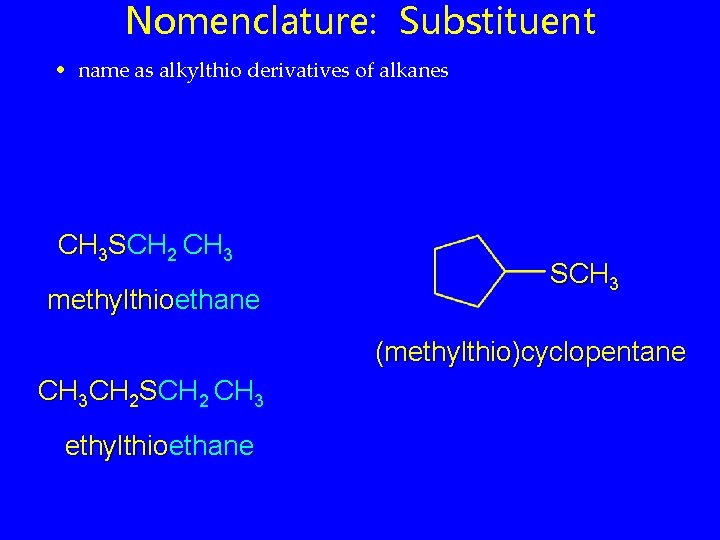
Nomenclature: Substituent • name as alkylthio derivatives of alkanes CH 3 SCH 2 CH 3 methylthioethane SCH 3 (methylthio)cyclopentane CH 3 CH 2 SCH 2 CH 3 ethylthioethane
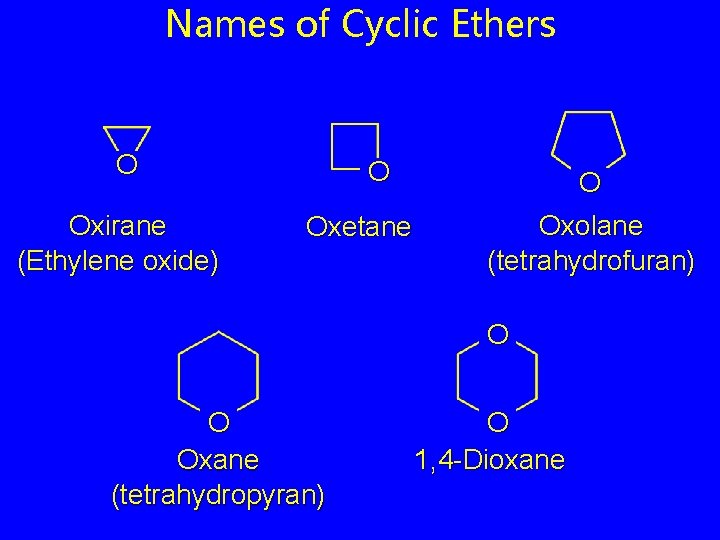
Names of Cyclic Ethers O Oxirane (Ethylene oxide) O Oxetane O Oxolane (tetrahydrofuran) O O Oxane (tetrahydropyran) O 1, 4 -Dioxane
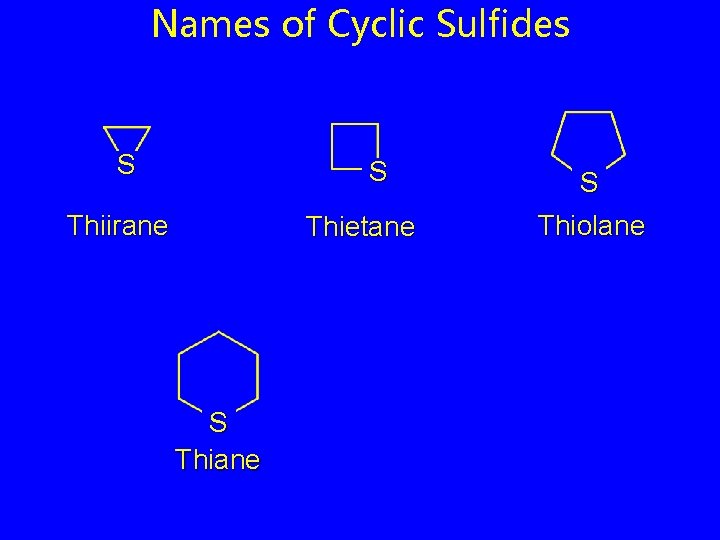
Names of Cyclic Sulfides S S Thiirane Thietane S Thiolane
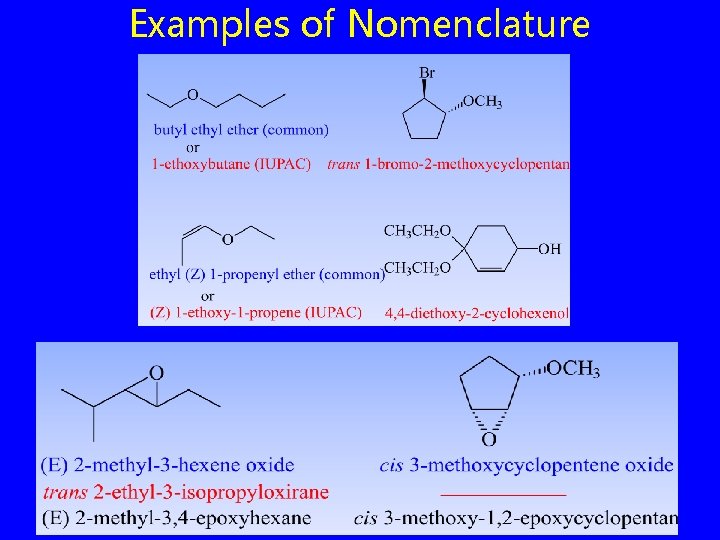
Examples of Nomenclature
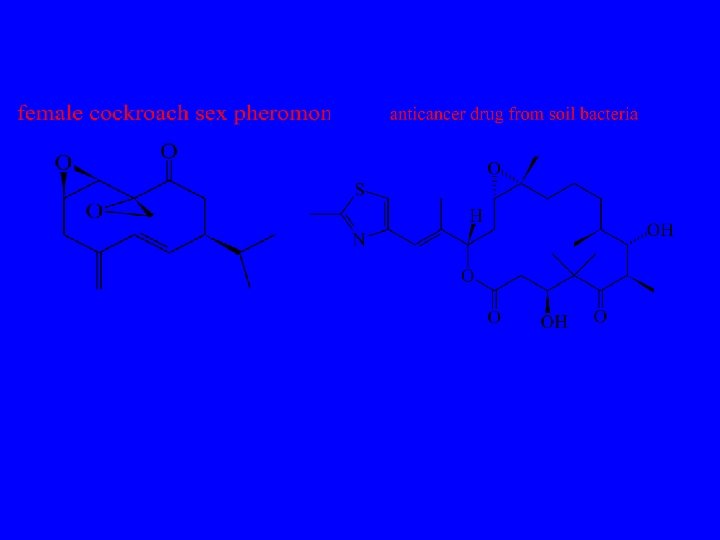
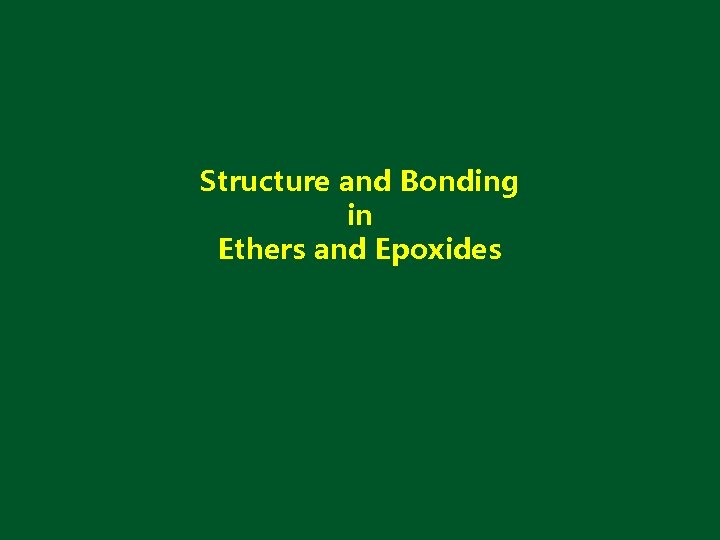
Structure and Bonding in Ethers and Epoxides
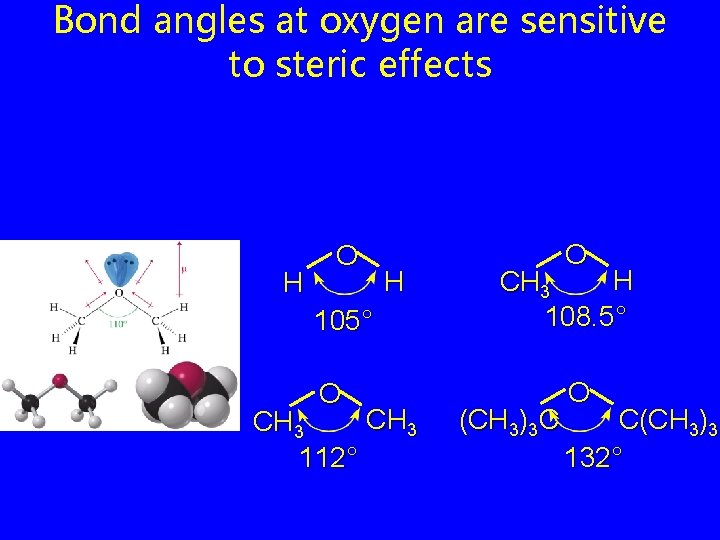
Bond angles at oxygen are sensitive to steric effects H O H 105° O CH 3 112° O H CH 3 108. 5° (CH 3)3 C O C(CH 3)3 132°
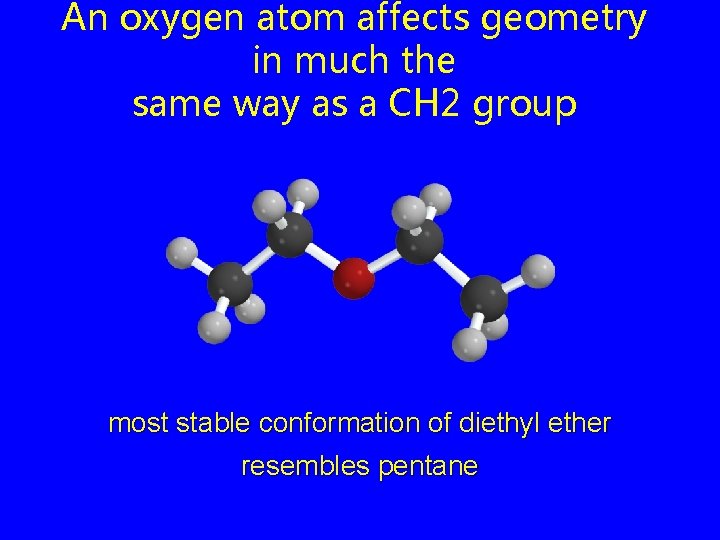
An oxygen atom affects geometry in much the same way as a CH 2 group most stable conformation of diethyl ether resembles pentane
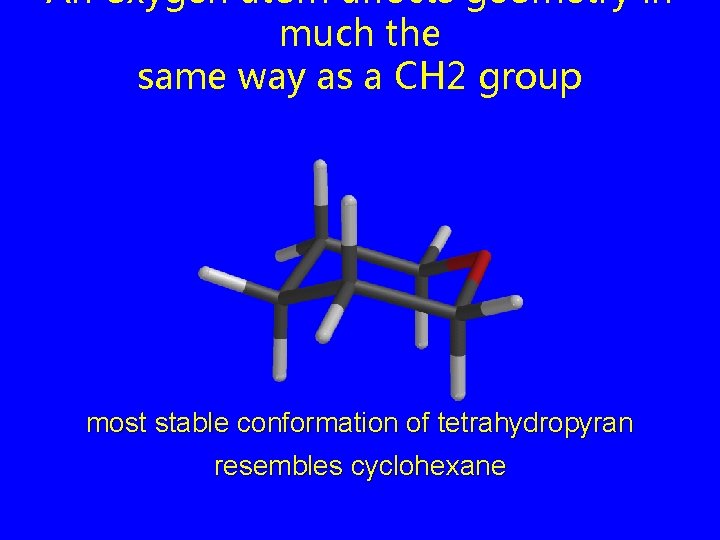
An oxygen atom affects geometry in much the same way as a CH 2 group most stable conformation of tetrahydropyran resembles cyclohexane
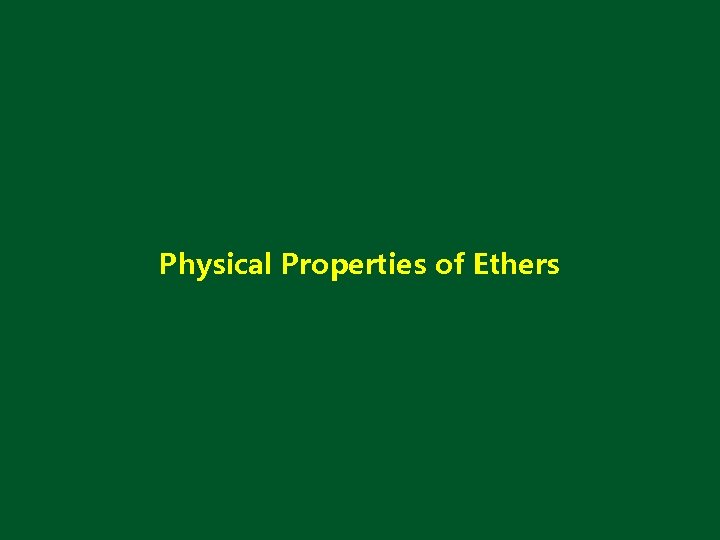
Physical Properties of Ethers
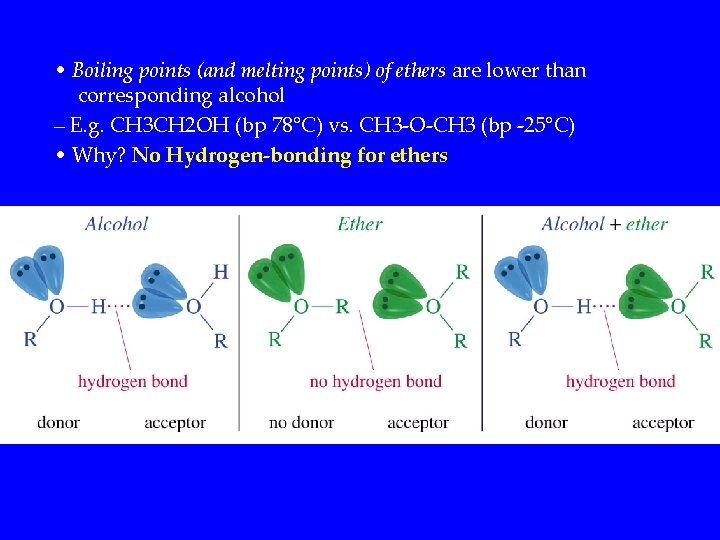
• Boiling points (and melting points) of ethers are lower than corresponding alcohol – E. g. CH 3 CH 2 OH (bp 78°C) vs. CH 3 -O-CH 3 (bp -25°C) • Why? No Hydrogen-bonding for ethers
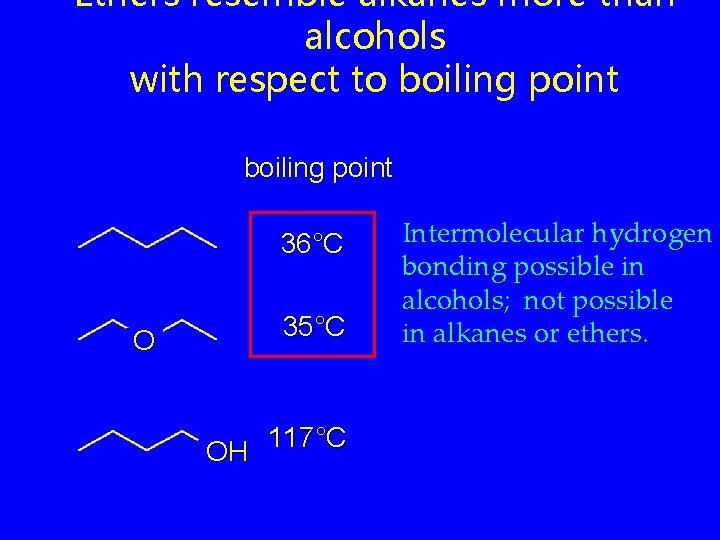
Ethers resemble alkanes more than alcohols with respect to boiling point 36°C O 35°C 117°C OH Intermolecular hydrogen bonding possible in alcohols; not possible in alkanes or ethers.
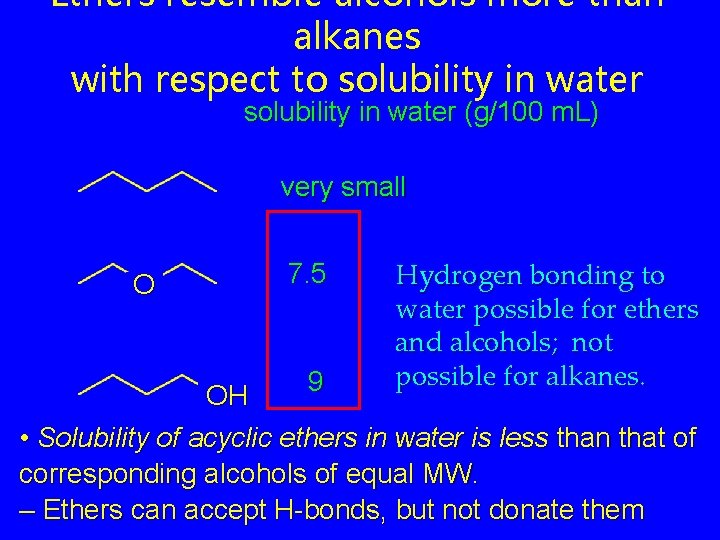
Ethers resemble alcohols more than alkanes with respect to solubility in water (g/100 m. L) very small O 7. 5 9 Hydrogen bonding to water possible for ethers and alcohols; not possible for alkanes. OH • Solubility of acyclic ethers in water is less than that of corresponding alcohols of equal MW. – Ethers can accept H-bonds, but not donate them
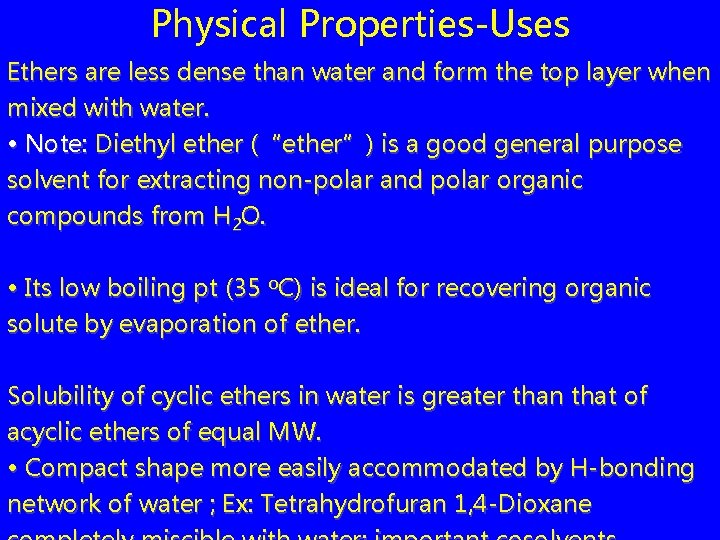
Physical Properties-Uses Ethers are less dense than water and form the top layer when mixed with water. • Note: Diethyl ether (“ether”) is a good general purpose solvent for extracting non-polar and polar organic compounds from H 2 O. • Its low boiling pt (35 o. C) is ideal for recovering organic solute by evaporation of ether. Solubility of cyclic ethers in water is greater than that of acyclic ethers of equal MW. • Compact shape more easily accommodated by H-bonding network of water ; Ex: Tetrahydrofuran 1, 4 -Dioxane
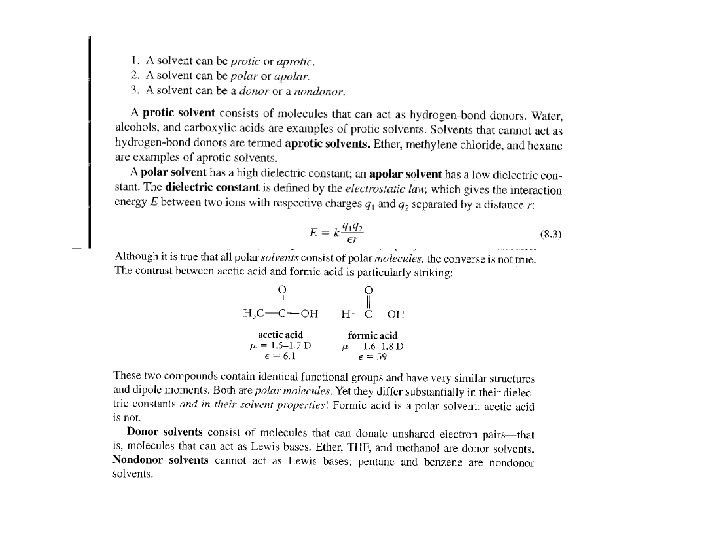
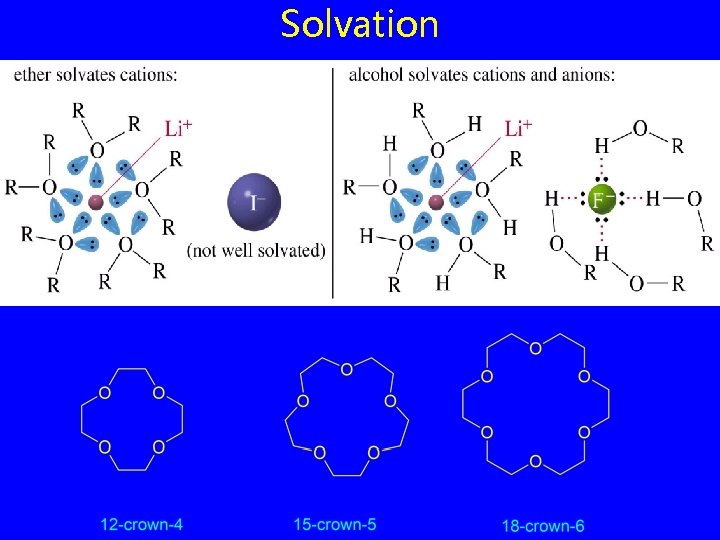
Solvation
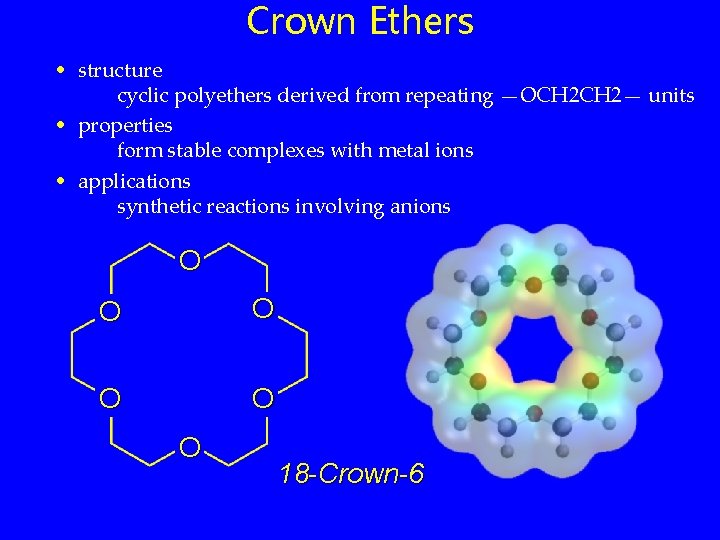
Crown Ethers • structure cyclic polyethers derived from repeating —OCH 2— units • properties form stable complexes with metal ions • applications synthetic reactions involving anions O O O 18 -Crown-6
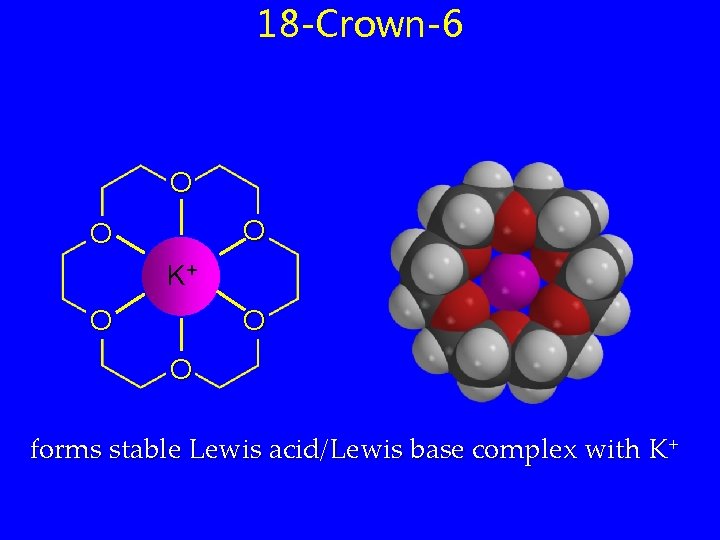
18 -Crown-6 O O O K+ O O O forms stable Lewis acid/Lewis base complex with K+
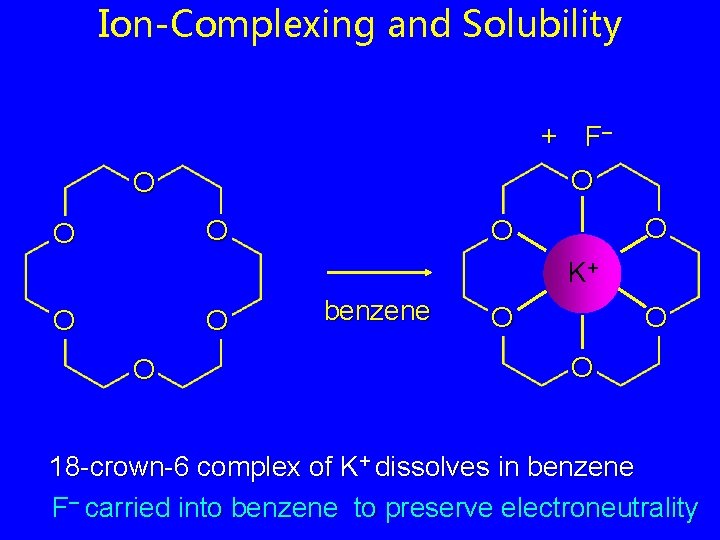
Ion-Complexing and Solubility + F– O O O K+ O O O benzene O O O 18 -crown-6 complex of K+ dissolves in benzene F– carried into benzene to preserve electroneutrality
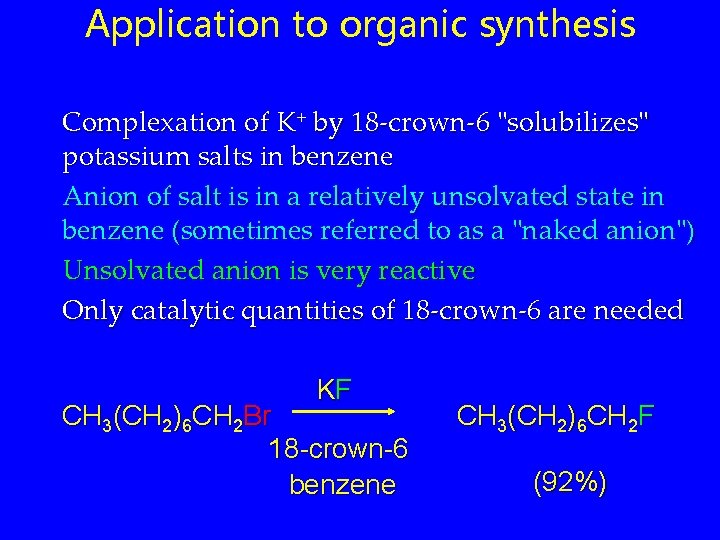
Application to organic synthesis Complexation of K+ by 18 -crown-6 "solubilizes" potassium salts in benzene Anion of salt is in a relatively unsolvated state in benzene (sometimes referred to as a "naked anion") Unsolvated anion is very reactive Only catalytic quantities of 18 -crown-6 are needed KF CH 3(CH 2)6 CH 2 Br 18 -crown-6 benzene CH 3(CH 2)6 CH 2 F (92%)
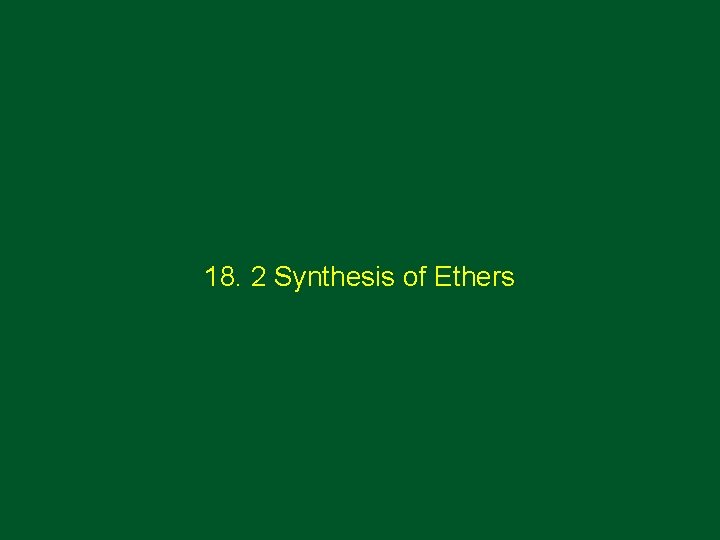
18. 2 Synthesis of Ethers
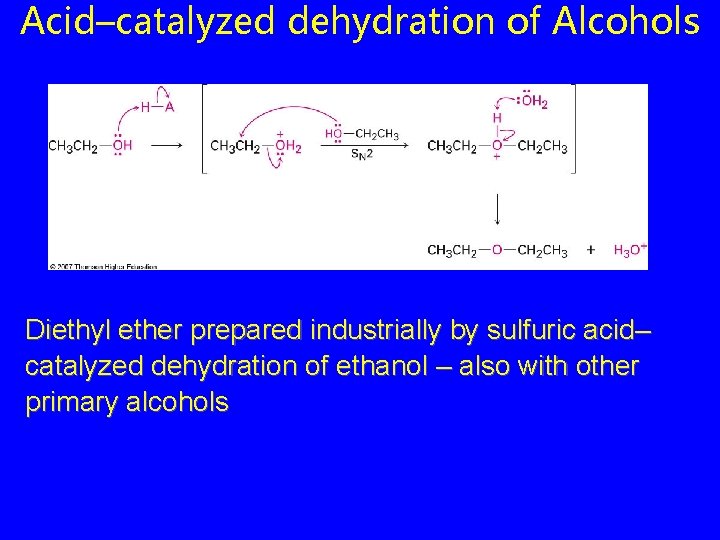
Acid–catalyzed dehydration of Alcohols Diethyl ether prepared industrially by sulfuric acid– catalyzed dehydration of ethanol – also with other primary alcohols
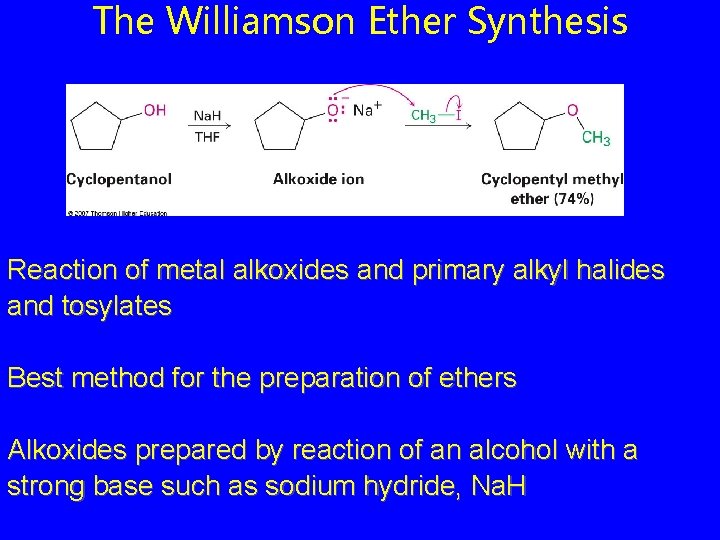
The Williamson Ether Synthesis Reaction of metal alkoxides and primary alkyl halides and tosylates Best method for the preparation of ethers Alkoxides prepared by reaction of an alcohol with a strong base such as sodium hydride, Na. H
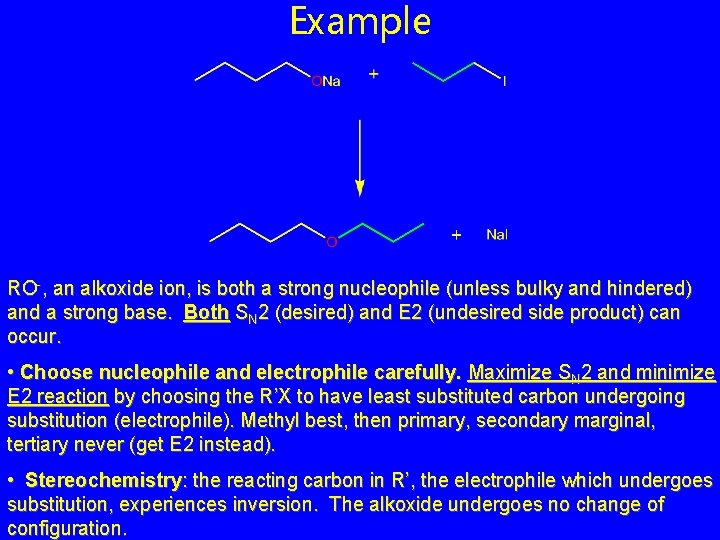
Example RO-, an alkoxide ion, is both a strong nucleophile (unless bulky and hindered) and a strong base. Both SN 2 (desired) and E 2 (undesired side product) can occur. • Choose nucleophile and electrophile carefully. Maximize SN 2 and minimize E 2 reaction by choosing the R’X to have least substituted carbon undergoing substitution (electrophile). Methyl best, then primary, secondary marginal, tertiary never (get E 2 instead). • Stereochemistry: the reacting carbon in R’, the electrophile which undergoes substitution, experiences inversion. The alkoxide undergoes no change of configuration.
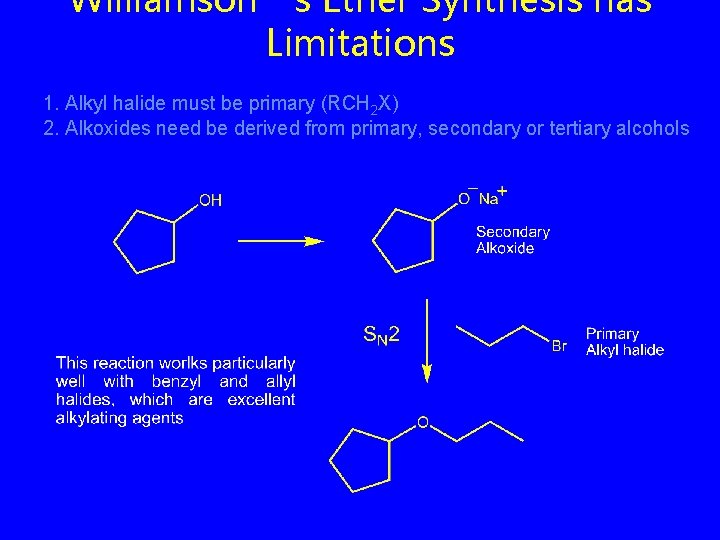
Williamson’s Ether Synthesis has Limitations 1. Alkyl halide must be primary (RCH 2 X) 2. Alkoxides need be derived from primary, secondary or tertiary alcohols
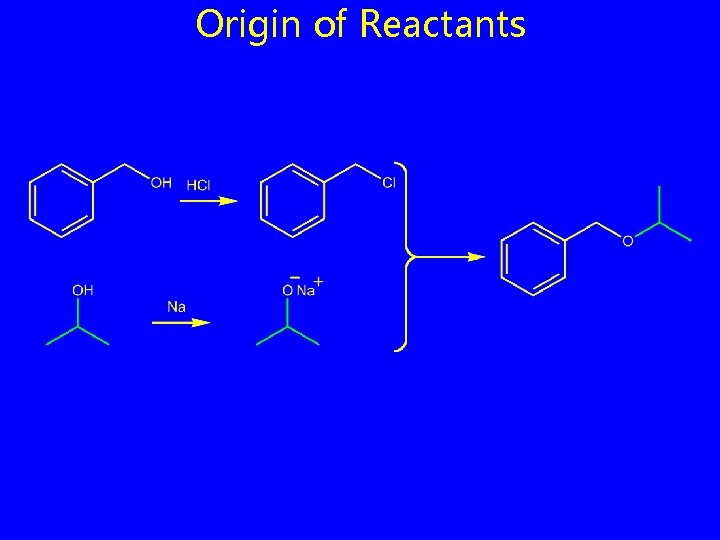
Origin of Reactants
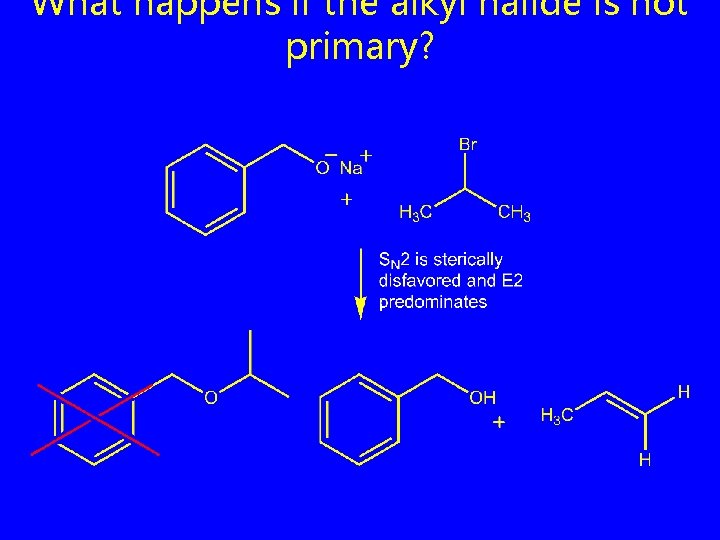
What happens if the alkyl halide is not primary?
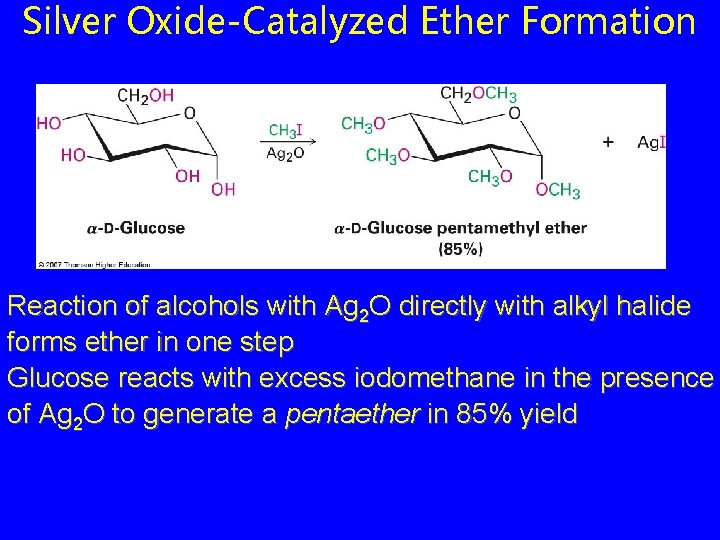
Silver Oxide-Catalyzed Ether Formation Reaction of alcohols with Ag 2 O directly with alkyl halide forms ether in one step Glucose reacts with excess iodomethane in the presence of Ag 2 O to generate a pentaether in 85% yield
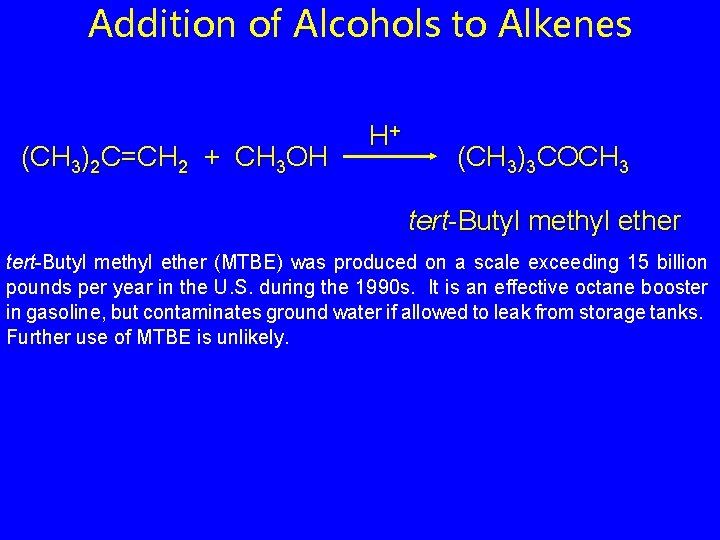
Addition of Alcohols to Alkenes (CH 3)2 C=CH 2 + CH 3 OH H+ (CH 3)3 COCH 3 tert-Butyl methyl ether (MTBE) was produced on a scale exceeding 15 billion pounds per year in the U. S. during the 1990 s. It is an effective octane booster in gasoline, but contaminates ground water if allowed to leak from storage tanks. Further use of MTBE is unlikely.
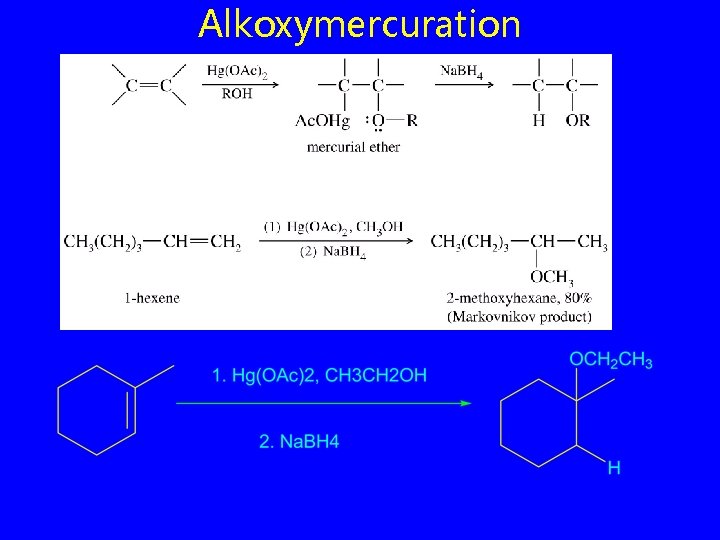
Alkoxymercuration
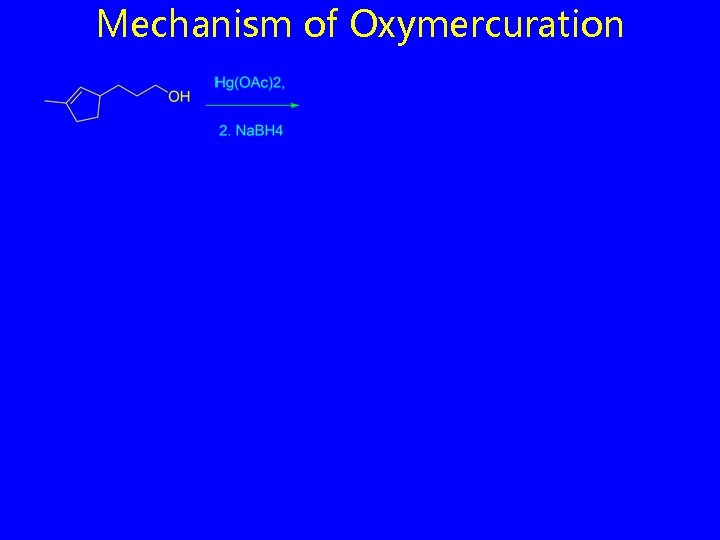
Mechanism of Oxymercuration
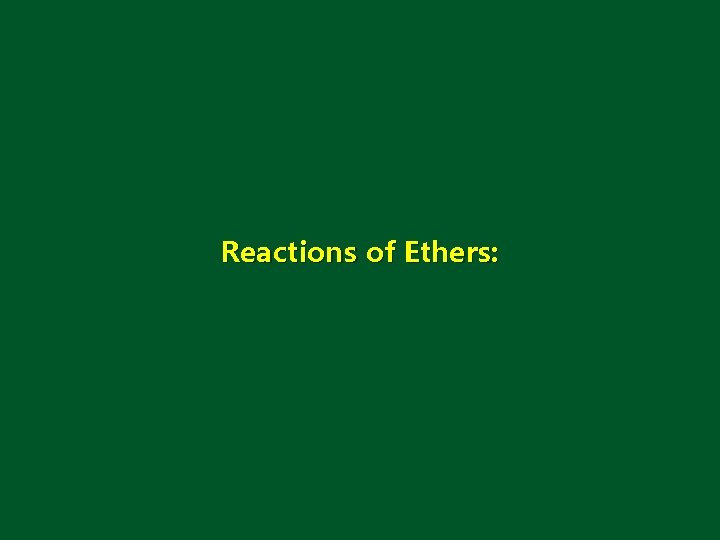
Reactions of Ethers:
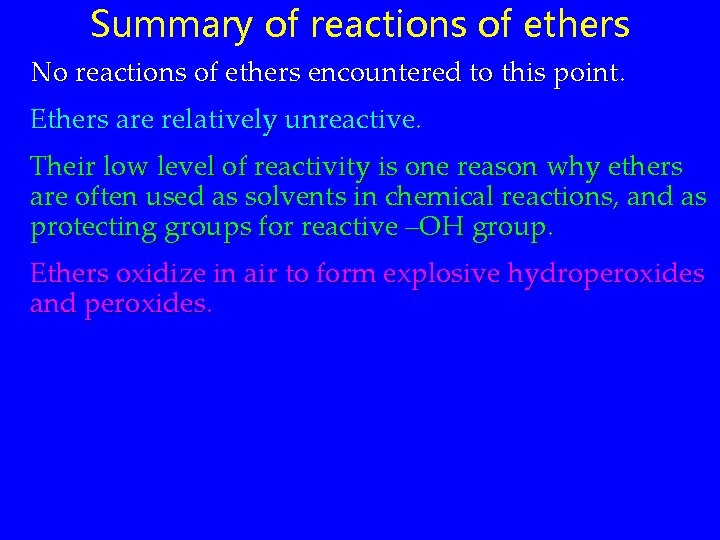
Summary of reactions of ethers No reactions of ethers encountered to this point. Ethers are relatively unreactive. Their low level of reactivity is one reason why ethers are often used as solvents in chemical reactions, and as protecting groups for reactive –OH group. Ethers oxidize in air to form explosive hydroperoxides and peroxides.
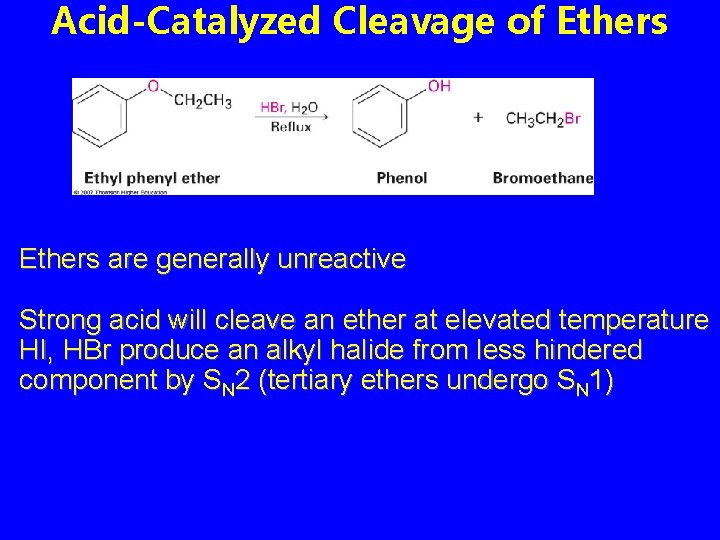
Acid-Catalyzed Cleavage of Ethers are generally unreactive Strong acid will cleave an ether at elevated temperature HI, HBr produce an alkyl halide from less hindered component by SN 2 (tertiary ethers undergo SN 1)
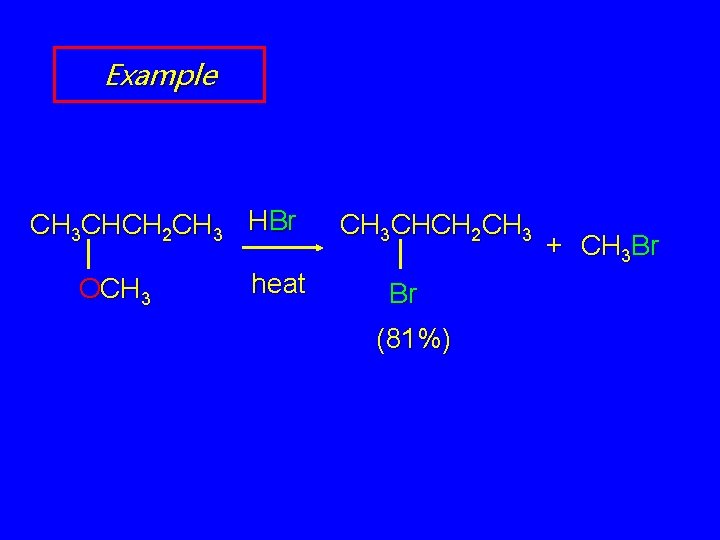
Example CH 3 CHCH 2 CH 3 HBr OCH 3 heat CH 3 CHCH 2 CH 3 Br (81%) + CH 3 Br
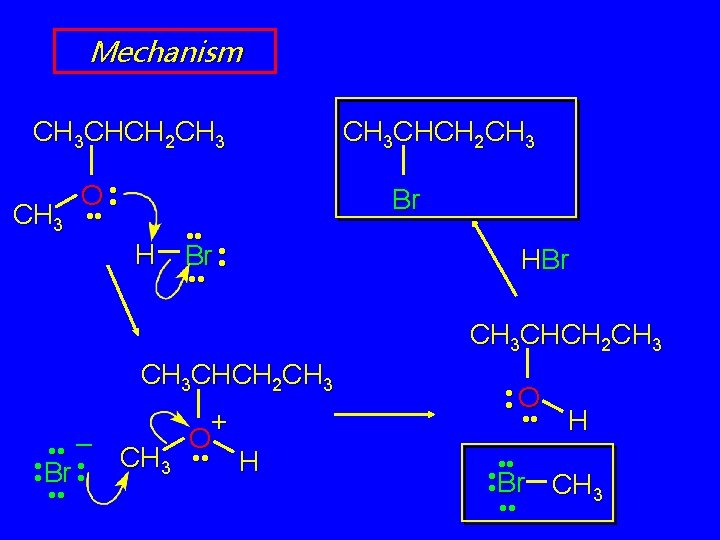
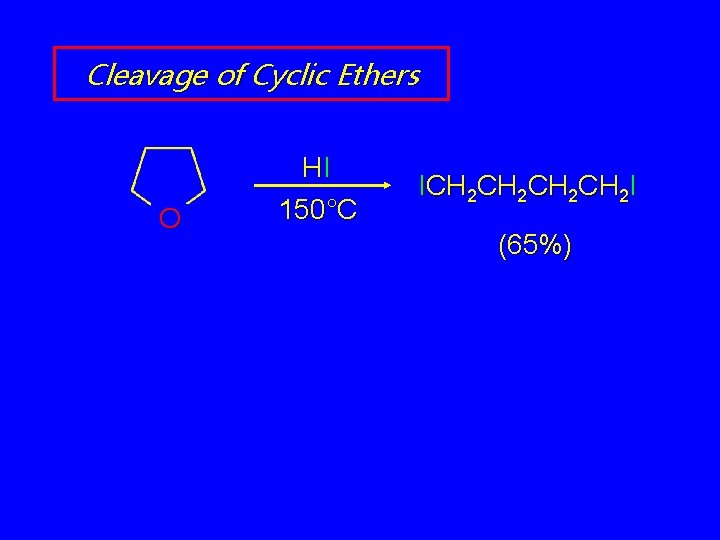
Cleavage of Cyclic Ethers O HI 150°C ICH 2 CH 2 I (65%)
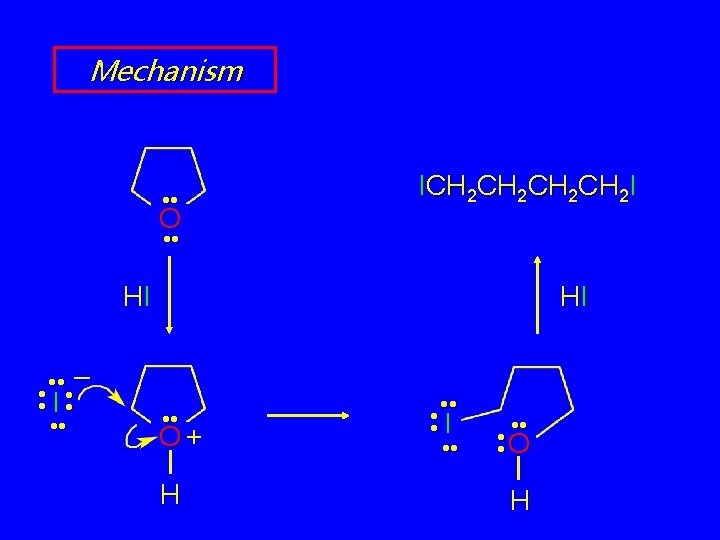
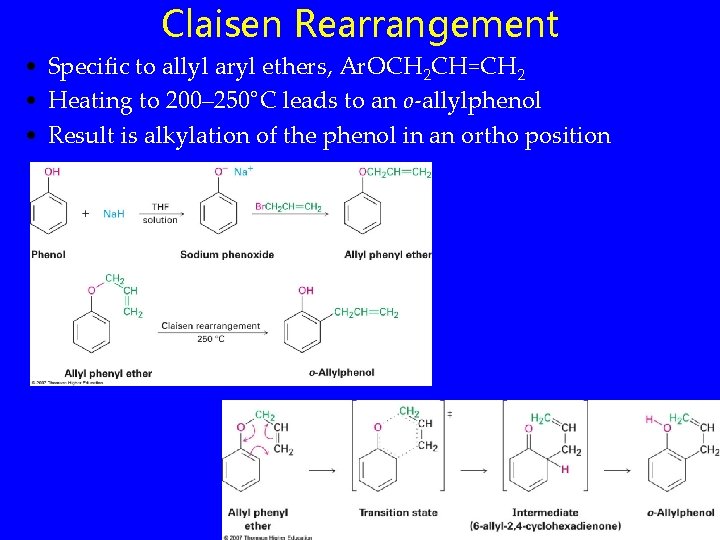
Claisen Rearrangement • Specific to allyl aryl ethers, Ar. OCH 2 CH=CH 2 • Heating to 200– 250°C leads to an o-allylphenol • Result is alkylation of the phenol in an ortho position
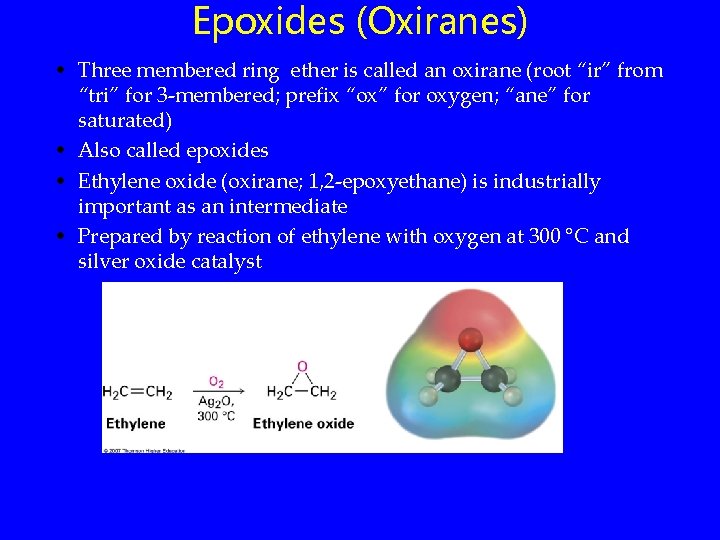
Epoxides (Oxiranes) • Three membered ring ether is called an oxirane (root “ir” from “tri” for 3 -membered; prefix “ox” for oxygen; “ane” for saturated) • Also called epoxides • Ethylene oxide (oxirane; 1, 2 -epoxyethane) is industrially important as an intermediate • Prepared by reaction of ethylene with oxygen at 300 °C and silver oxide catalyst
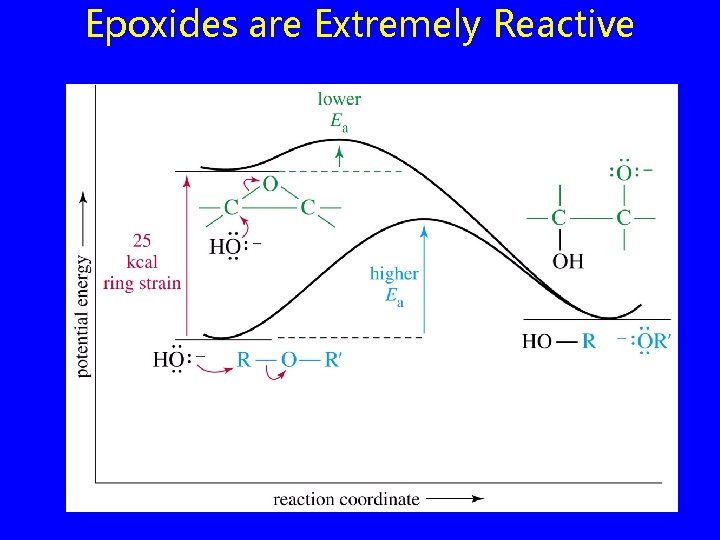
Epoxides are Extremely Reactive
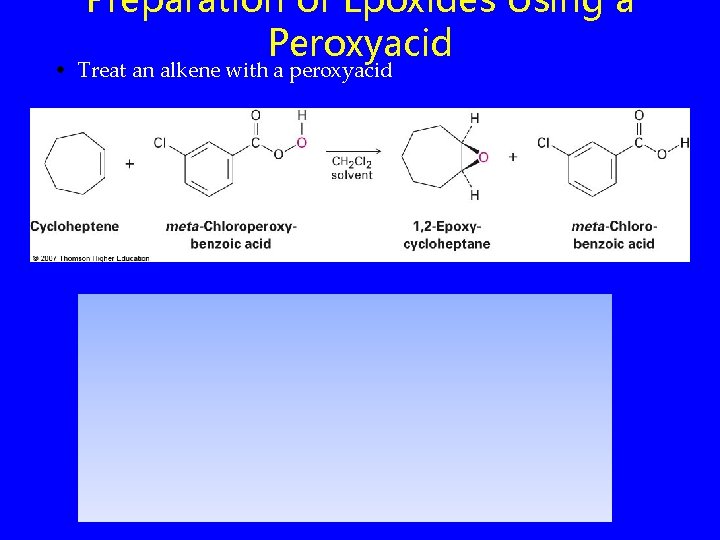
Preparation of Epoxides Using a Peroxyacid • Treat an alkene with a peroxyacid
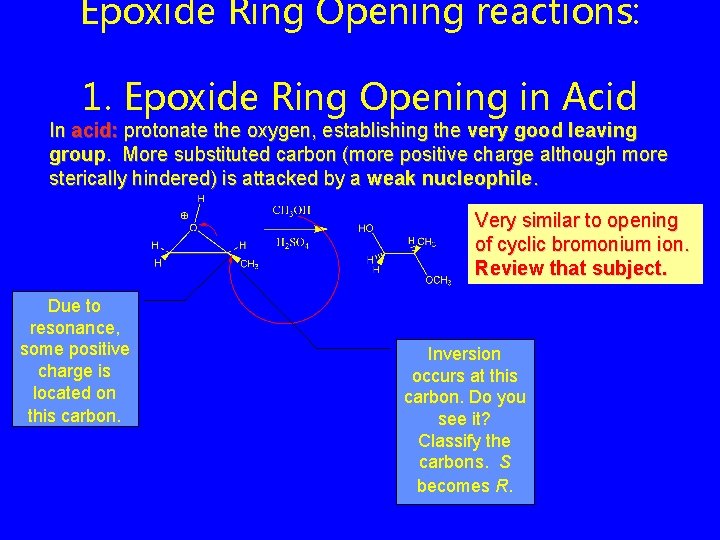
Epoxide Ring Opening reactions: 1. Epoxide Ring Opening in Acid In acid: protonate the oxygen, establishing the very good leaving group. More substituted carbon (more positive charge although more sterically hindered) is attacked by a weak nucleophile. Very similar to opening of cyclic bromonium ion. Review that subject. Due to resonance, some positive charge is located on this carbon. Inversion occurs at this carbon. Do you see it? Classify the carbons. S becomes R.
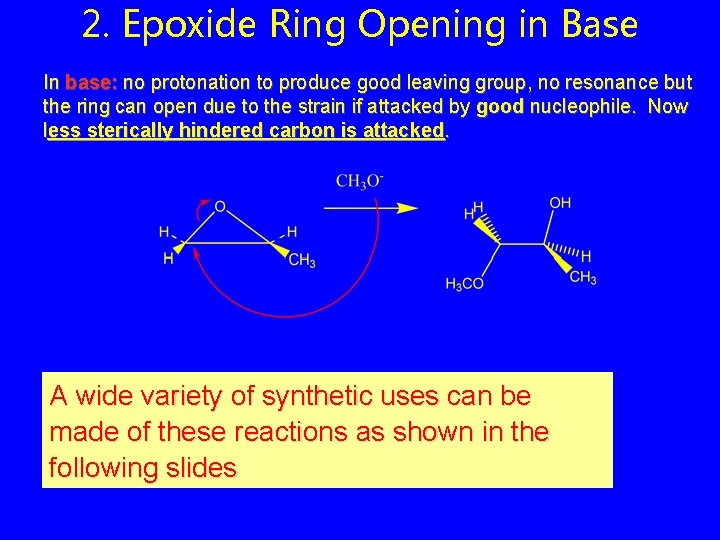
2. Epoxide Ring Opening in Base In base: no protonation to produce good leaving group, no resonance but the ring can open due to the strain if attacked by good nucleophile. Now less sterically hindered carbon is attacked. A wide variety of synthetic uses can be made of these reactions as shown in the following slides
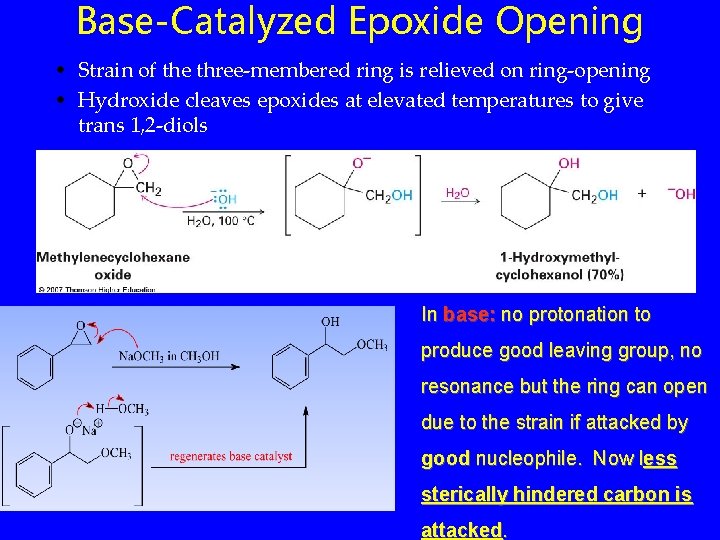
Base-Catalyzed Epoxide Opening • Strain of the three-membered ring is relieved on ring-opening • Hydroxide cleaves epoxides at elevated temperatures to give trans 1, 2 -diols In base: no protonation to produce good leaving group, no resonance but the ring can open due to the strain if attacked by good nucleophile. Now less sterically hindered carbon is attacked.
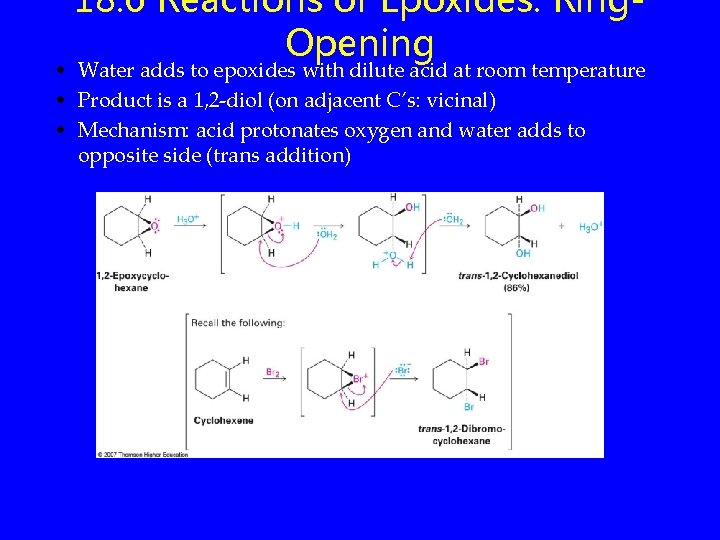
18. 6 Reactions of Epoxides: Ring. Opening • Water adds to epoxides with dilute acid at room temperature • Product is a 1, 2 -diol (on adjacent C’s: vicinal) • Mechanism: acid protonates oxygen and water adds to opposite side (trans addition)
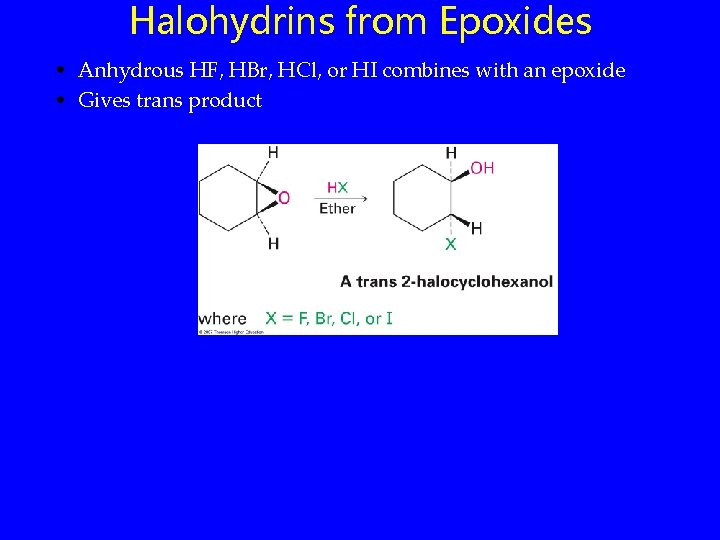
Halohydrins from Epoxides • Anhydrous HF, HBr, HCl, or HI combines with an epoxide • Gives trans product
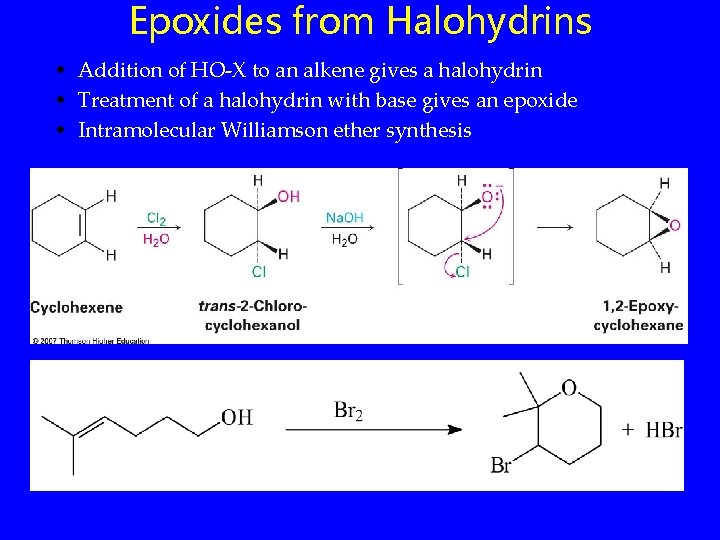
Epoxides from Halohydrins • Addition of HO-X to an alkene gives a halohydrin • Treatment of a halohydrin with base gives an epoxide • Intramolecular Williamson ether synthesis
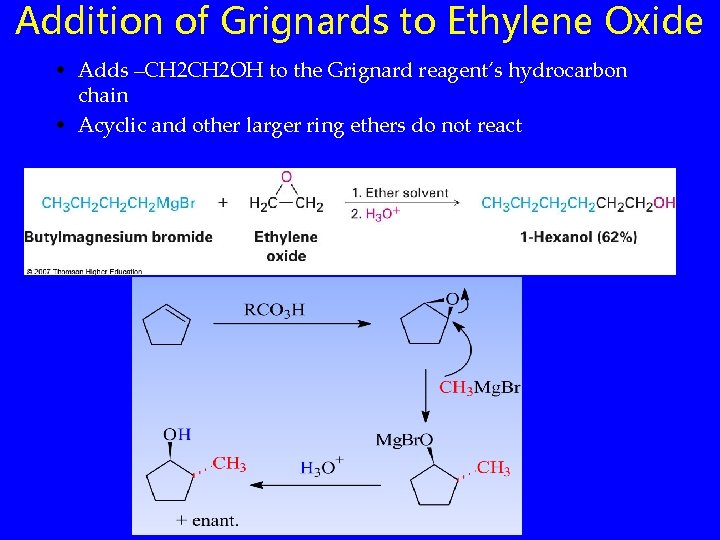
Addition of Grignards to Ethylene Oxide • Adds –CH 2 OH to the Grignard reagent’s hydrocarbon chain • Acyclic and other larger ring ethers do not react
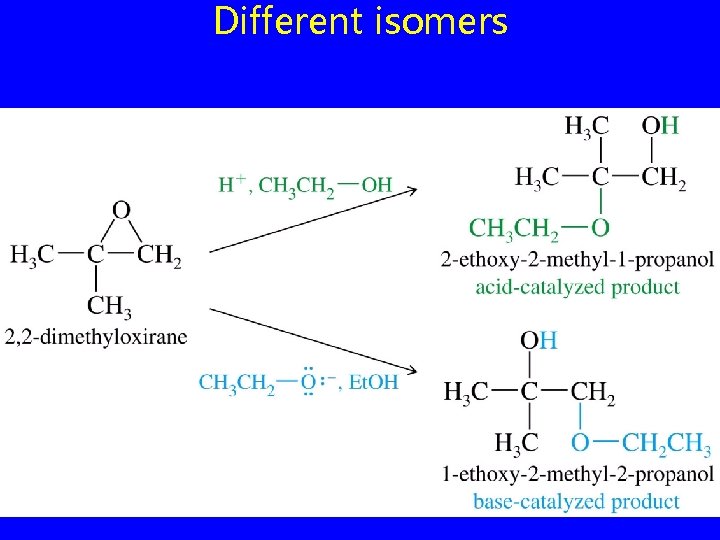
Different isomers
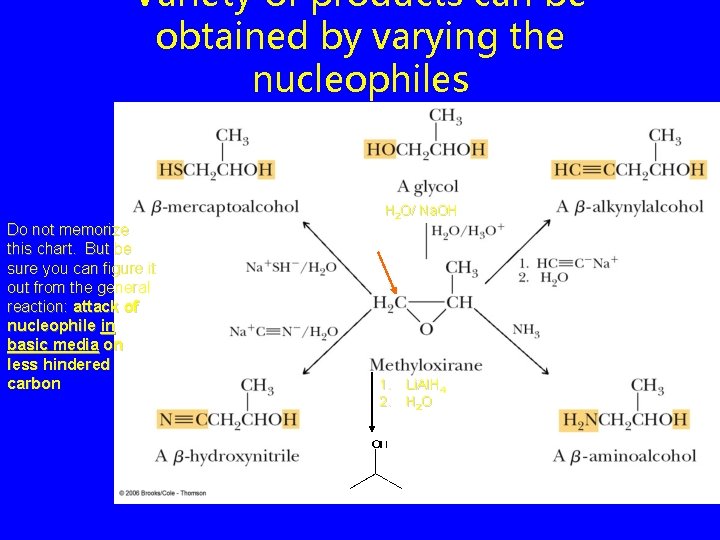
Variety of products can be obtained by varying the nucleophiles Do not memorize this chart. But be sure you can figure it out from the general reaction: attack of nucleophile in basic media on less hindered carbon H 2 O/ Na. OH 1. Li. Al. H 4 2. H 2 O
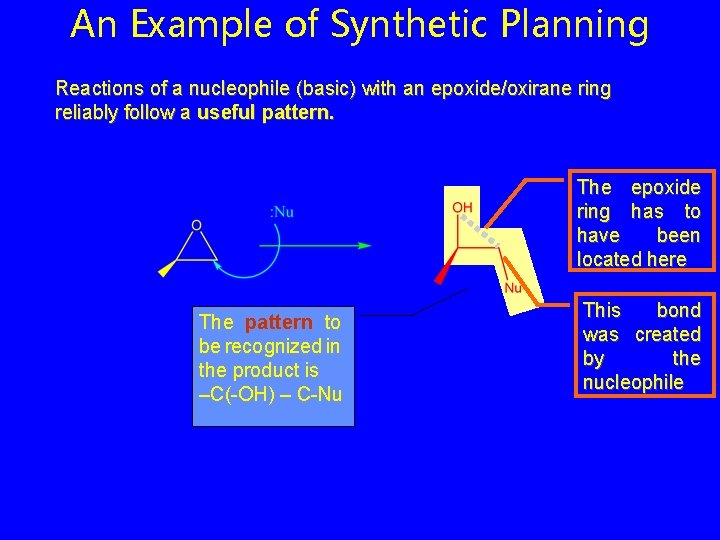
An Example of Synthetic Planning Reactions of a nucleophile (basic) with an epoxide/oxirane ring reliably follow a useful pattern. The epoxide ring has to have been located here The pattern to be recognized in the product is –C(-OH) – C-Nu This bond was created by the nucleophile
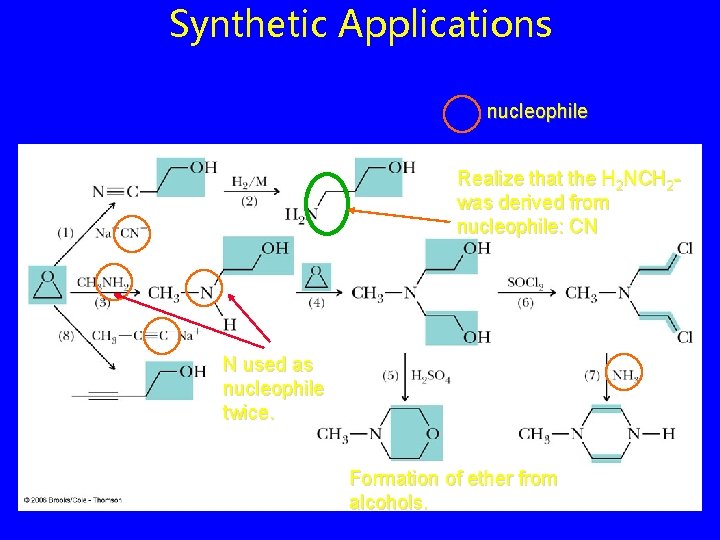
Synthetic Applications nucleophile Realize that the H 2 NCH 2 was derived from nucleophile: CN N used as nucleophile twice. Formation of ether from alcohols.
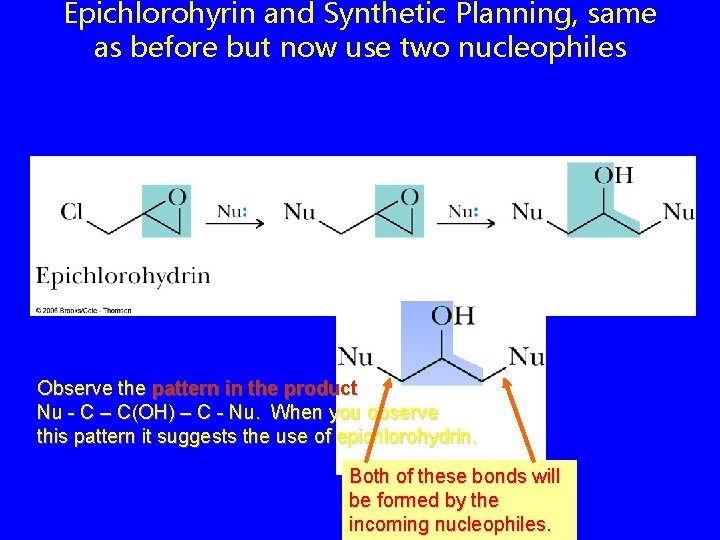
Epichlorohyrin and Synthetic Planning, same as before but now use two nucleophiles Observe the pattern in the product Nu - C – C(OH) – C - Nu. When you observe this pattern it suggests the use of epichlorohydrin. Both of these bonds will be formed by the incoming nucleophiles.
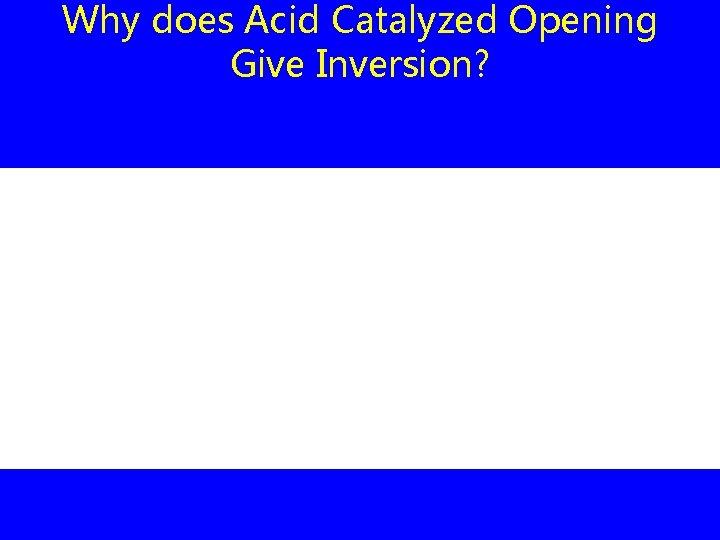
Why does Acid Catalyzed Opening Give Inversion?
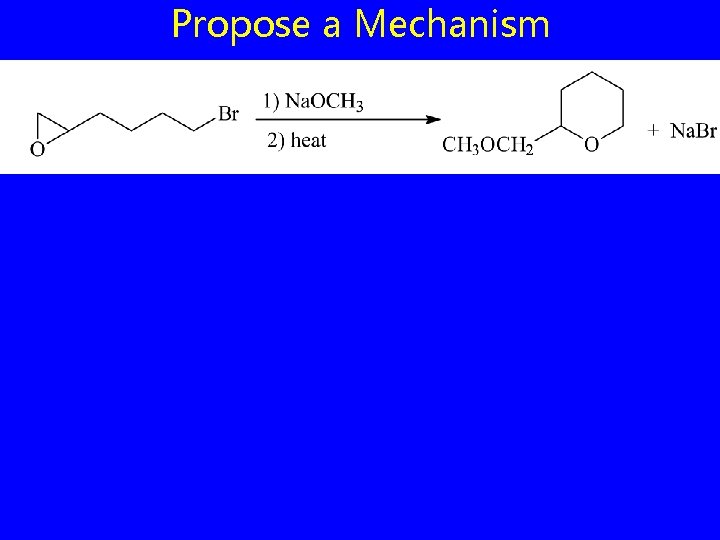
Propose a Mechanism
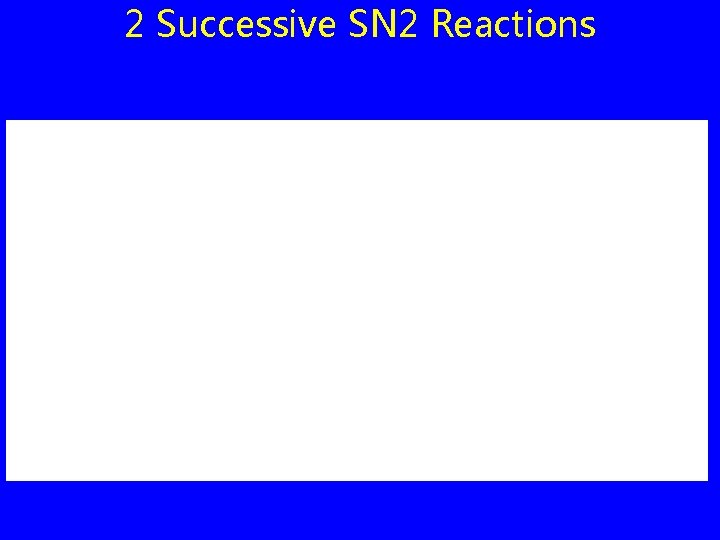
2 Successive SN 2 Reactions
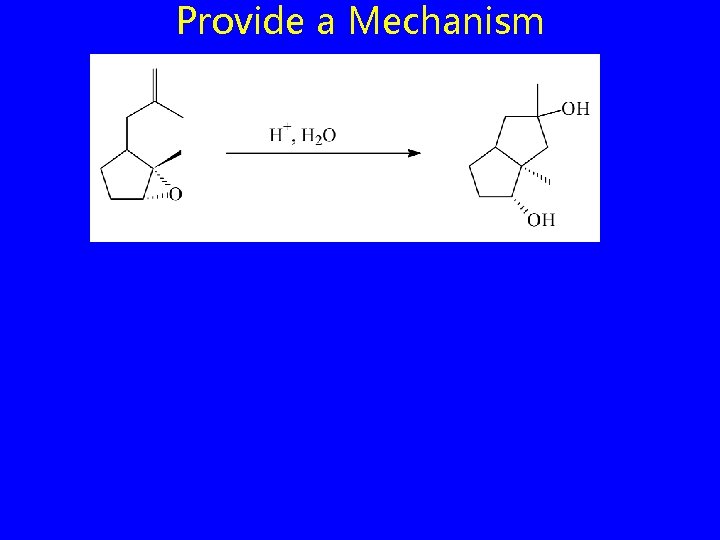
Provide a Mechanism
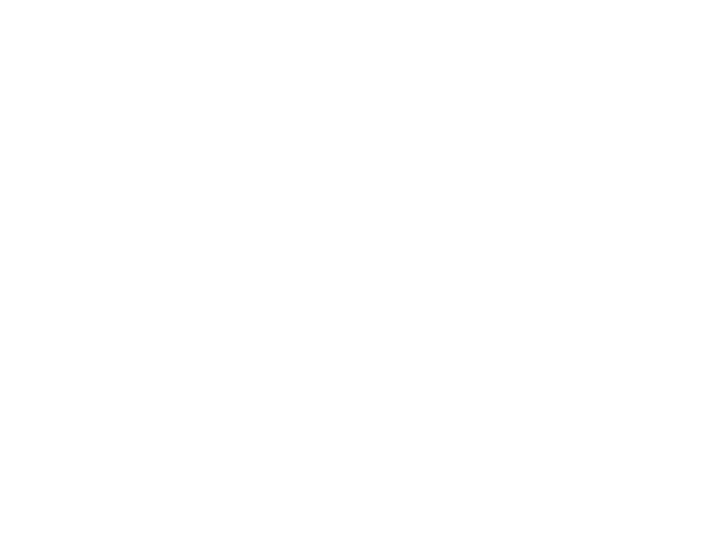
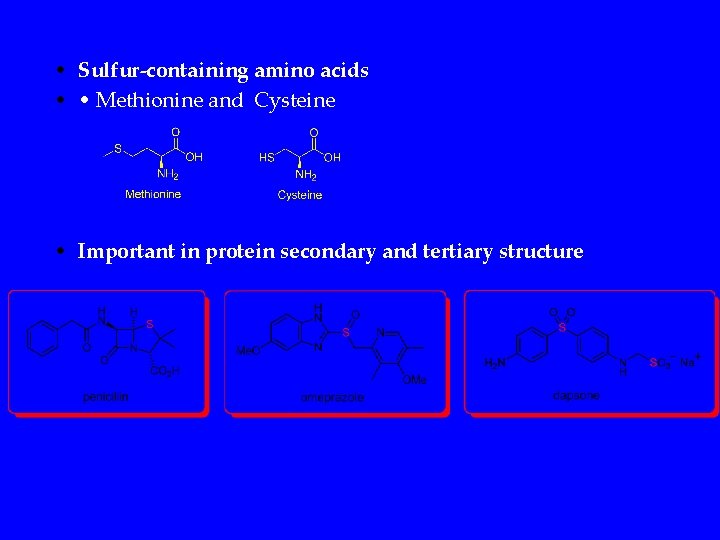
• Sulfur-containing amino acids • • Methionine and Cysteine • Important in protein secondary and tertiary structure
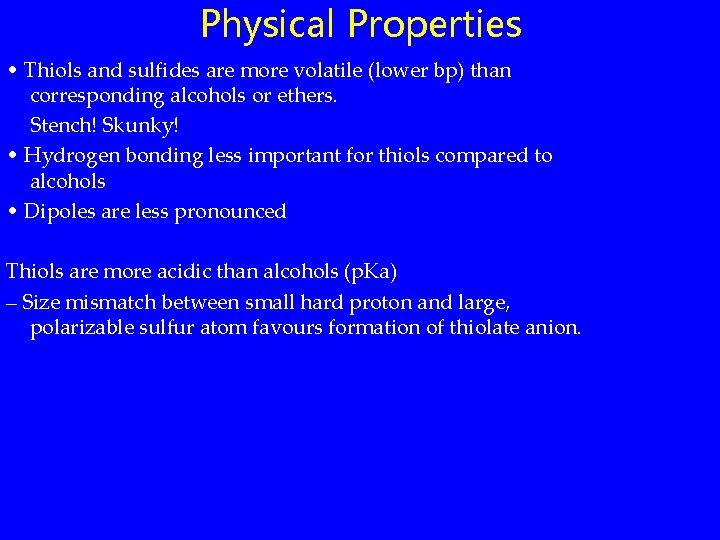
Physical Properties • Thiols and sulfides are more volatile (lower bp) than corresponding alcohols or ethers. Stench! Skunky! • Hydrogen bonding less important for thiols compared to alcohols • Dipoles are less pronounced Thiols are more acidic than alcohols (p. Ka) – Size mismatch between small hard proton and large, polarizable sulfur atom favours formation of thiolate anion.
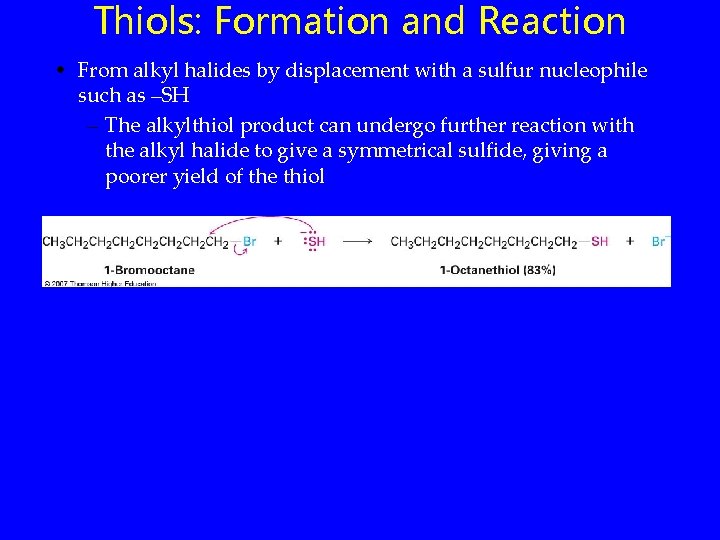
Thiols: Formation and Reaction • From alkyl halides by displacement with a sulfur nucleophile such as –SH – The alkylthiol product can undergo further reaction with the alkyl halide to give a symmetrical sulfide, giving a poorer yield of the thiol
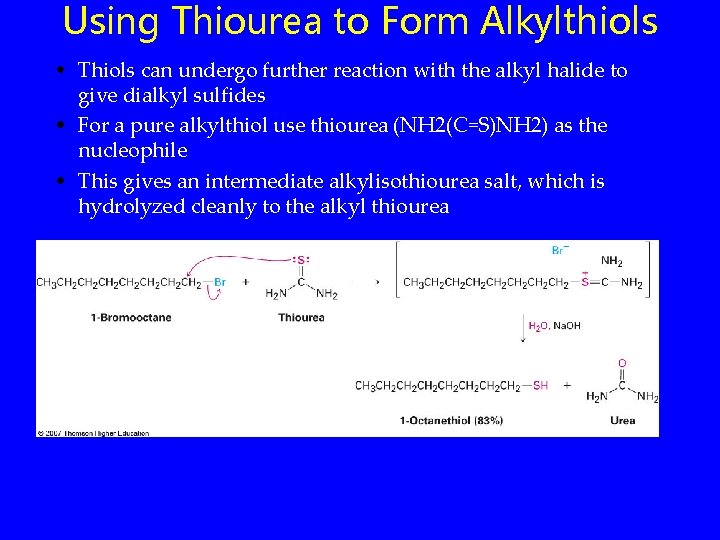
Using Thiourea to Form Alkylthiols • Thiols can undergo further reaction with the alkyl halide to give dialkyl sulfides • For a pure alkylthiol use thiourea (NH 2(C=S)NH 2) as the nucleophile • This gives an intermediate alkylisothiourea salt, which is hydrolyzed cleanly to the alkyl thiourea
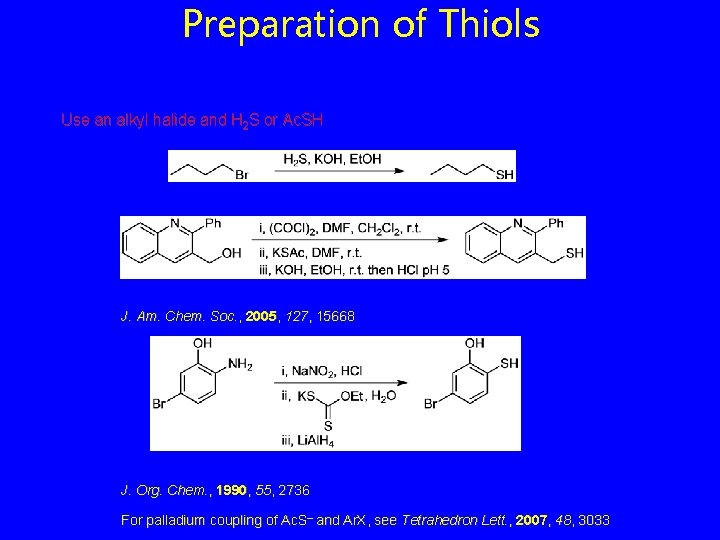
Preparation of Thiols Use an alkyl halide and H 2 S or Ac. SH J. Am. Chem. Soc. , 2005, 127, 15668 J. Org. Chem. , 1990, 55, 2736 For palladium coupling of Ac. S– and Ar. X, see Tetrahedron Lett. , 2007, 48, 3033
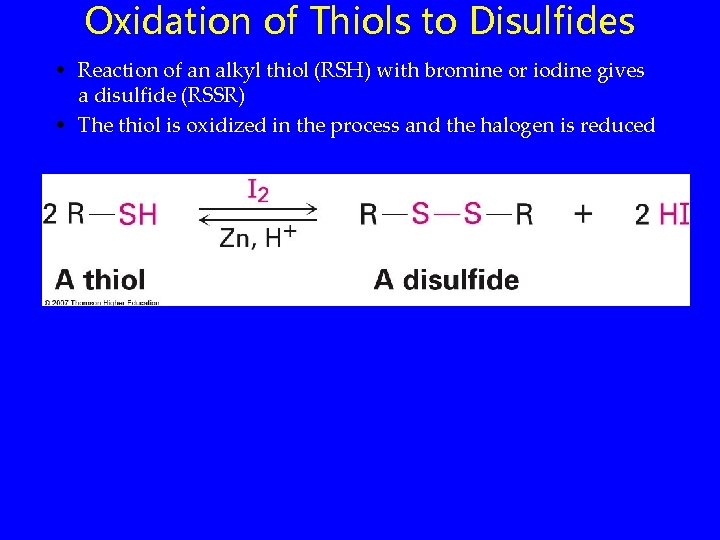
Oxidation of Thiols to Disulfides • Reaction of an alkyl thiol (RSH) with bromine or iodine gives a disulfide (RSSR) • The thiol is oxidized in the process and the halogen is reduced
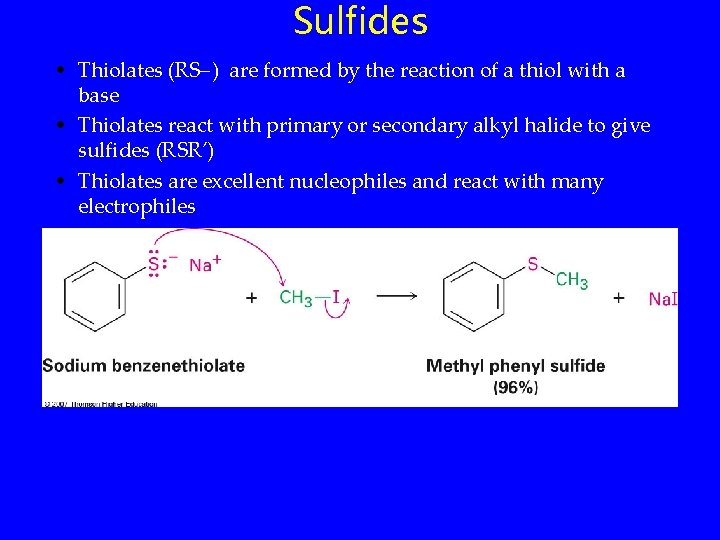
Sulfides • Thiolates (RS ) are formed by the reaction of a thiol with a base • Thiolates react with primary or secondary alkyl halide to give sulfides (RSR’) • Thiolates are excellent nucleophiles and react with many electrophiles
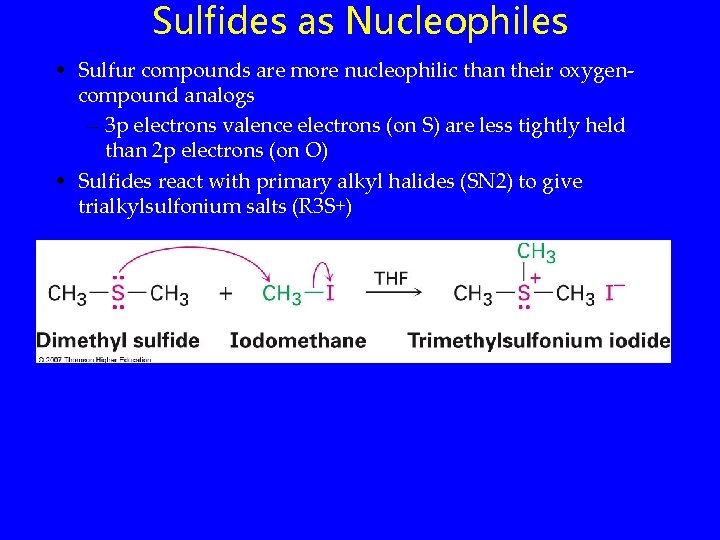
Sulfides as Nucleophiles • Sulfur compounds are more nucleophilic than their oxygencompound analogs – 3 p electrons valence electrons (on S) are less tightly held than 2 p electrons (on O) • Sulfides react with primary alkyl halides (SN 2) to give trialkylsulfonium salts (R 3 S+)
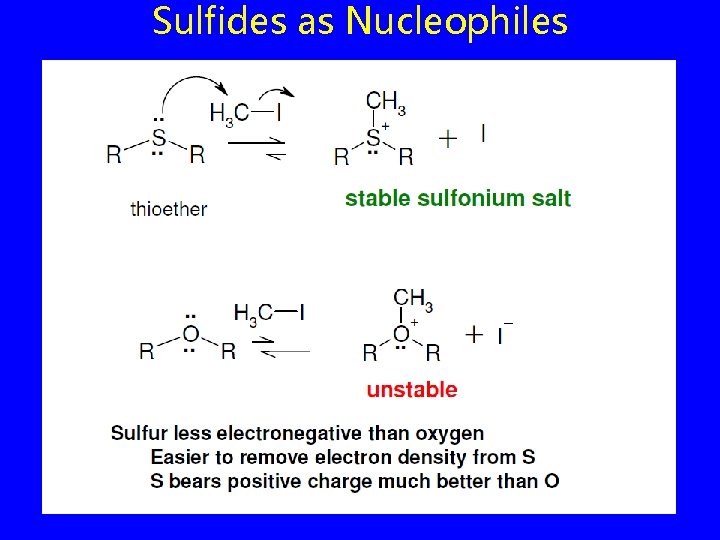
Sulfides as Nucleophiles
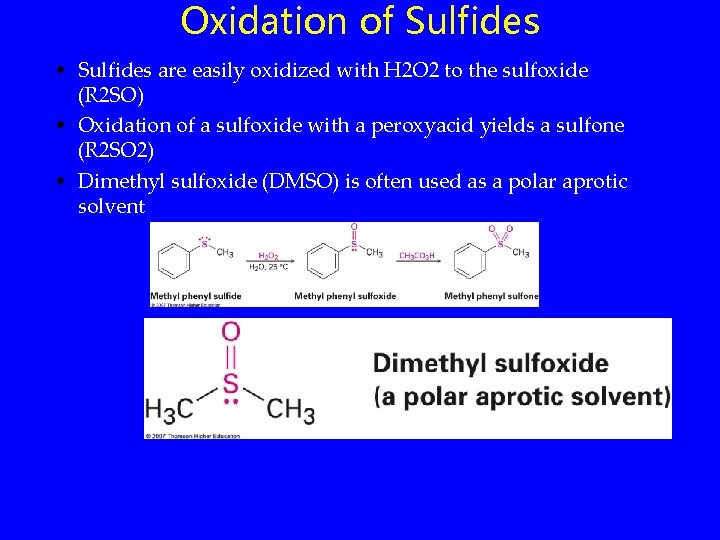
Oxidation of Sulfides • Sulfides are easily oxidized with H 2 O 2 to the sulfoxide (R 2 SO) • Oxidation of a sulfoxide with a peroxyacid yields a sulfone (R 2 SO 2) • Dimethyl sulfoxide (DMSO) is often used as a polar aprotic solvent
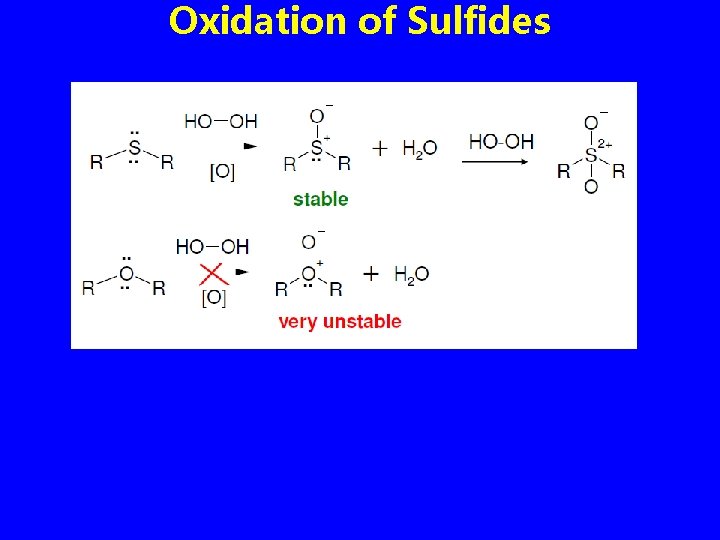
Oxidation of Sulfides
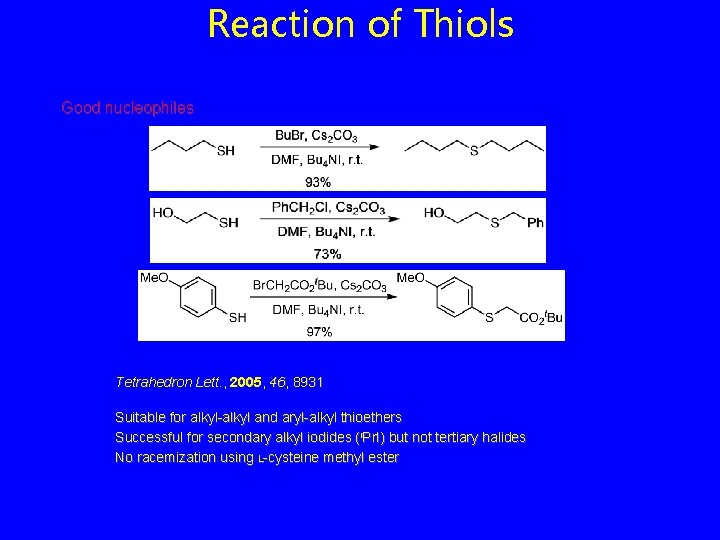
Reaction of Thiols Good nucleophiles Tetrahedron Lett. , 2005, 46, 8931 Suitable for alkyl-alkyl and aryl-alkyl thioethers Successful for secondary alkyl iodides (i. Pr. I) but not tertiary halides No racemization using L-cysteine methyl ester
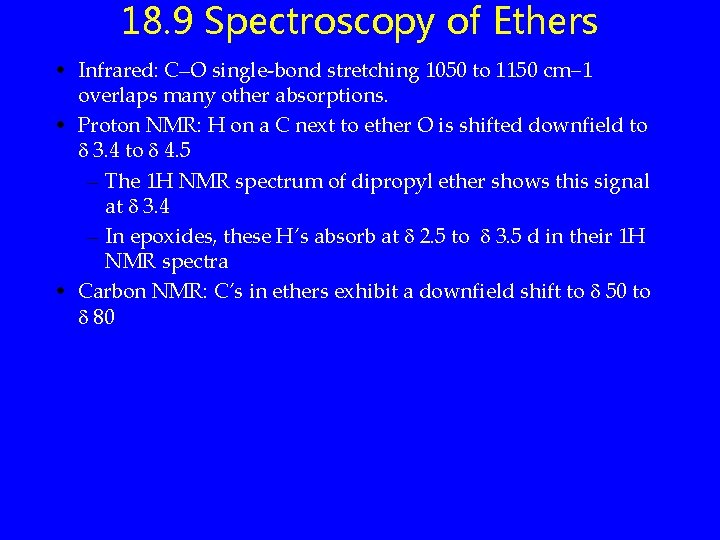
18. 9 Spectroscopy of Ethers • Infrared: C–O single-bond stretching 1050 to 1150 cm 1 overlaps many other absorptions. • Proton NMR: H on a C next to ether O is shifted downfield to 3. 4 to 4. 5 – The 1 H NMR spectrum of dipropyl ether shows this signal at 3. 4 – In epoxides, these H’s absorb at 2. 5 to 3. 5 d in their 1 H NMR spectra • Carbon NMR: C’s in ethers exhibit a downfield shift to 50 to 80
Alcohols phenols thiols and ethers
Hoch3 functional group
Nomenclature of ether
Upac name
Acidity of thiols
Ioc revelation thiols
Butaanzuur
Esters naming
Ether naming
Alcohols nomenclature
Preparation of ethers
Ethers boiling point
Organic chemistry david klein 3rd edition
Organic chemistry william h brown
Why are ethers relatively inert compounds
Acidic cleavage of ethers
Orange purple black
And because i am happy and dance and sing
West side story, romeo and juliet comparison chart
Taller and younger and shorter and older
5 centavos symbol
Indigo and violet colors
Gabby and sydney bought some pens
Young and dyslexic structure analysis
Albany movement
West yorkshire health and care partnership
Draw and label transverse wave
Smkvqmuzwv4 -site:youtube.com
Input independent variable
Transitive and intransitive and linking verbs
Define the term life role
Maintenance of reports and records
Transportation and assignment problems
Compare and contrast cold wave and wind chill factor
Final account format
Lesson preparation siop
Tomorrow and tomorrow
The ritz carlton motto
Ron offutt house
Muscles name
The great gatsby chapter 3 questions and answers
The giver chapter 3 quiz
Explanatory vs expository
Essay about similarities and differences
Compare and contrast analog and digital forecasting
The boy in the striped pyjamas film study
The blind man and the elephant poem questions
Disadvantages of deregulation
Venn diagram of typhoon and tornado
Form design in system analysis and design
Compare and contrast rainsford and zaroff venn diagram
Subject and simple subject
Compound inequality and
What is brazing in welding
Similes about summer
Is there a similarity between the parents and offspring
Separation of powers and checks and balances
Security program and policies principles and practices
Local maximum and minimum vs. absolute maximum and minimum
Megan and ron ate too much
Rounding numbers rules
The theme of hate in romeo and juliet
Protagonist and antagonist in romeo and juliet
Summary of act 4 and 5 romeo and juliet
Tricky dicky richard nixon
Three of a kind all letting fly
Remains poem simon armitage
Non terminating decimal
About rainbow
Jekyll and hyde psychoanalysis
Transverse and longitudinal waves both *
Production planning and inventory control
Present simple present progressive past simple
Foamy flake meaning
Trend and plunge notation
Pits and fissures definition
Compare and contrast physical and chemical changes
What is the difference between ncbts and ppst
Perbezaan copy and paste dan cut and paste
Serratus attachments