The mechanisms of flame quenching through porous media
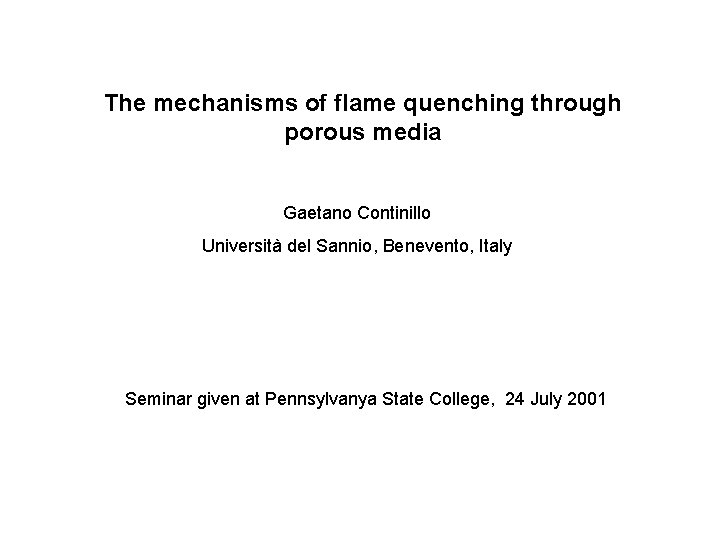
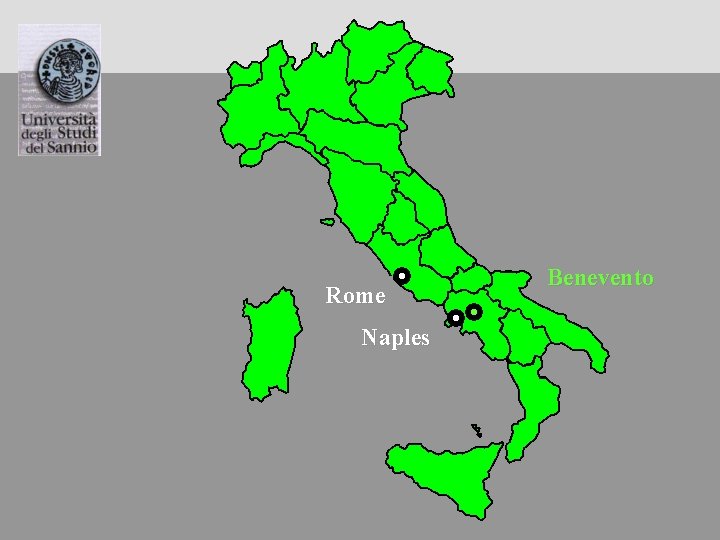
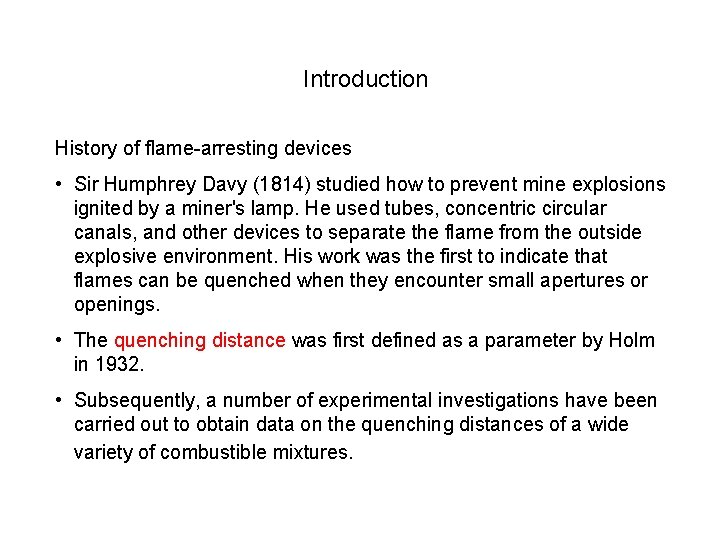
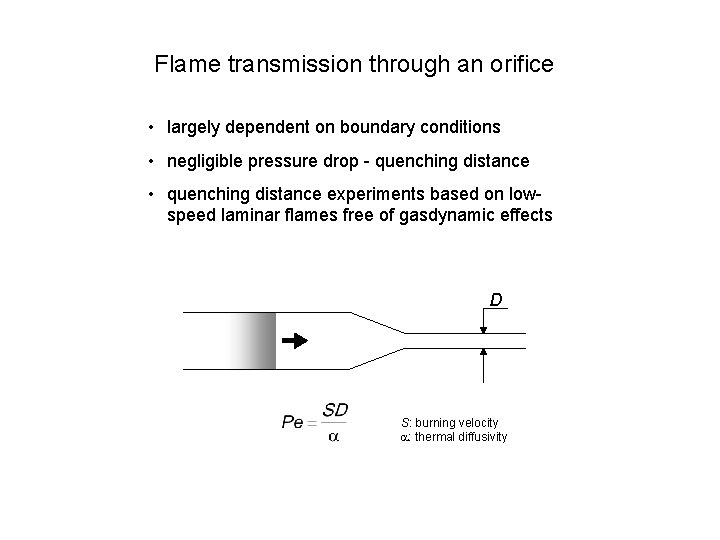
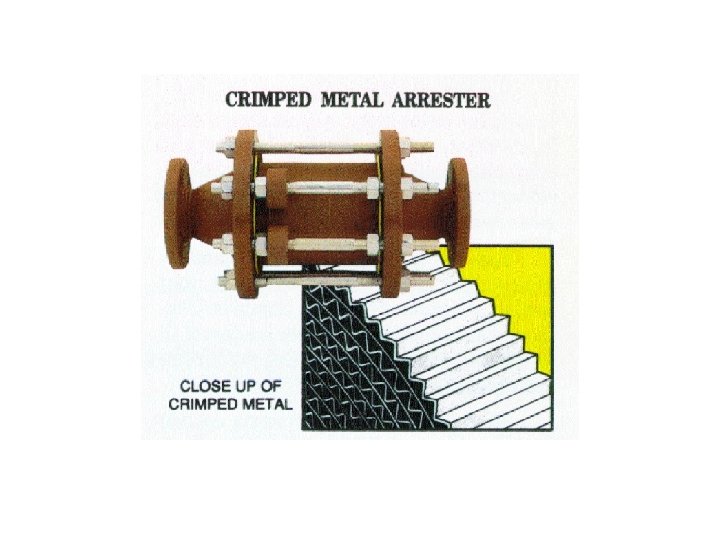
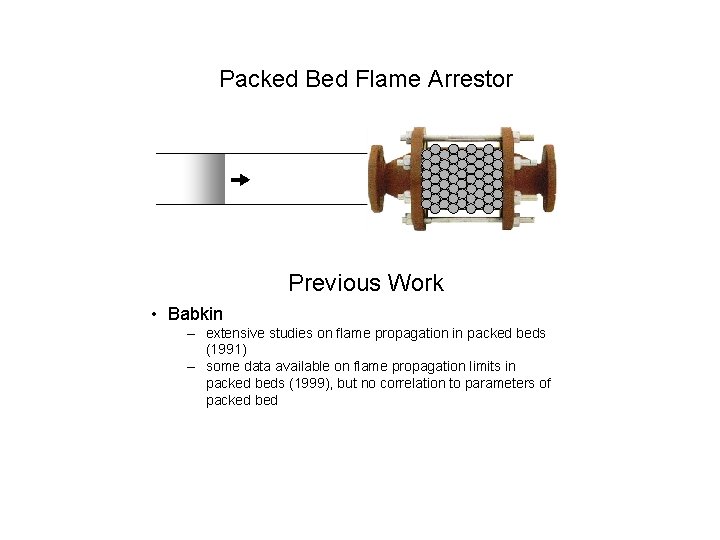
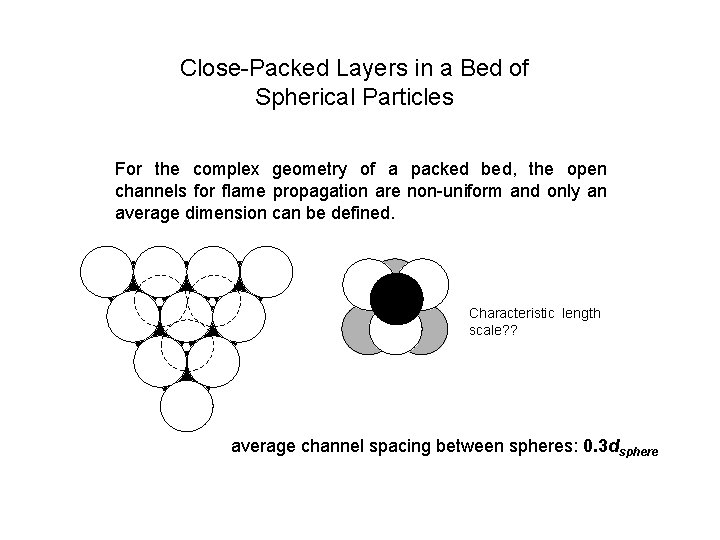
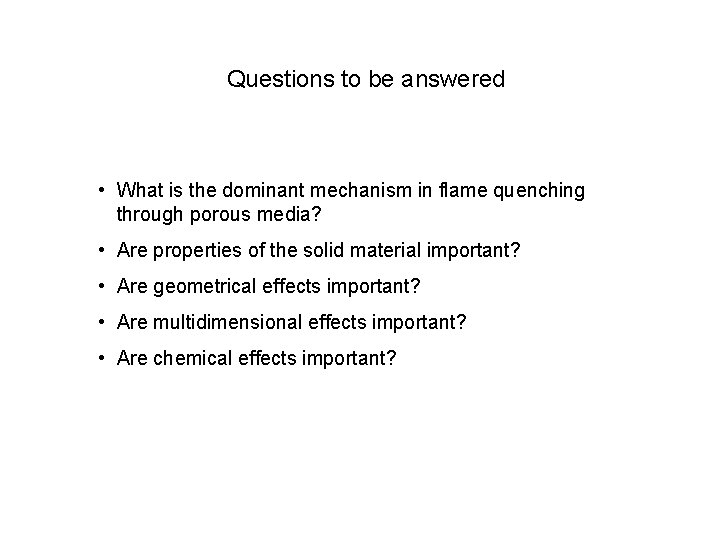
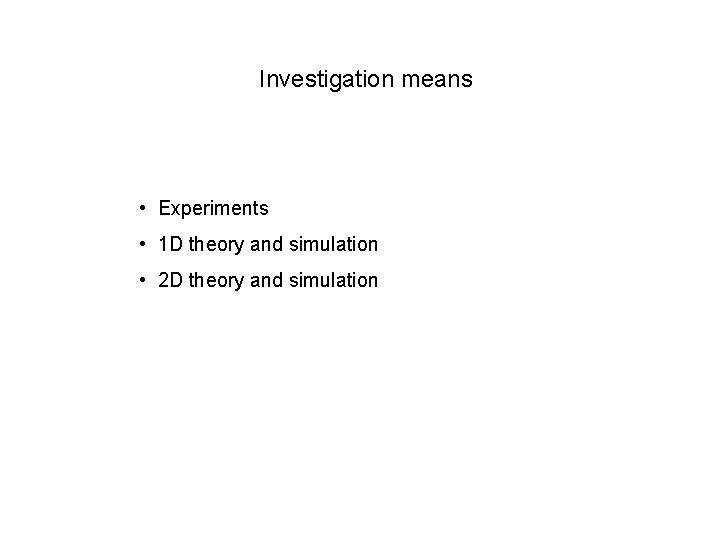
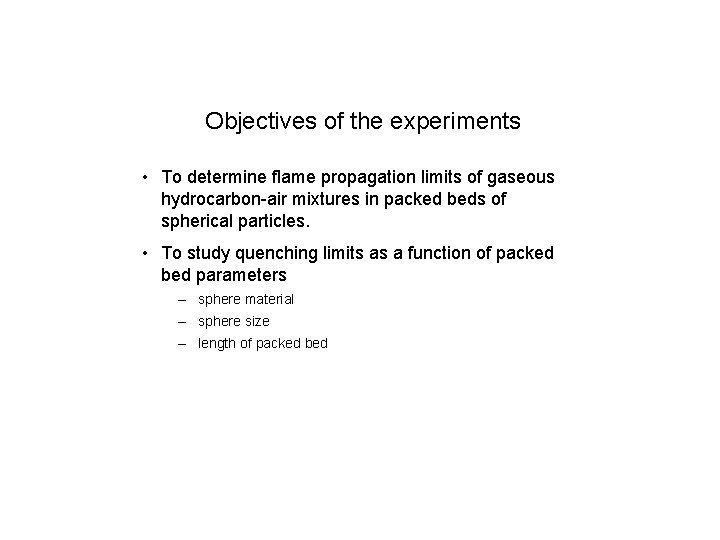
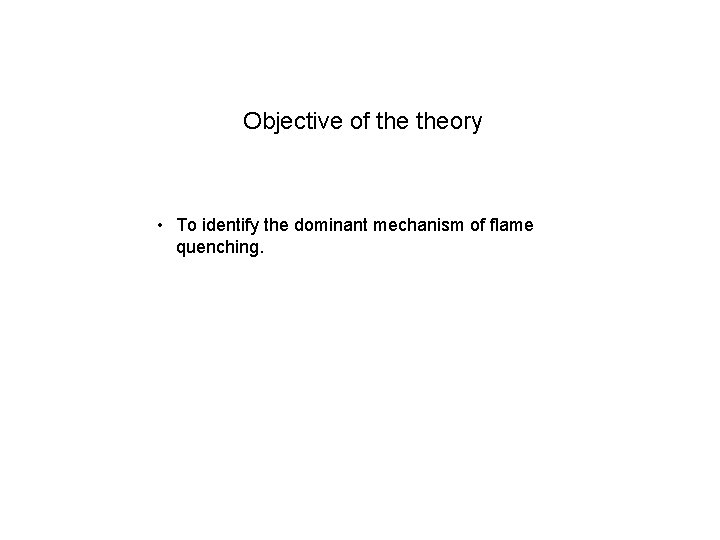
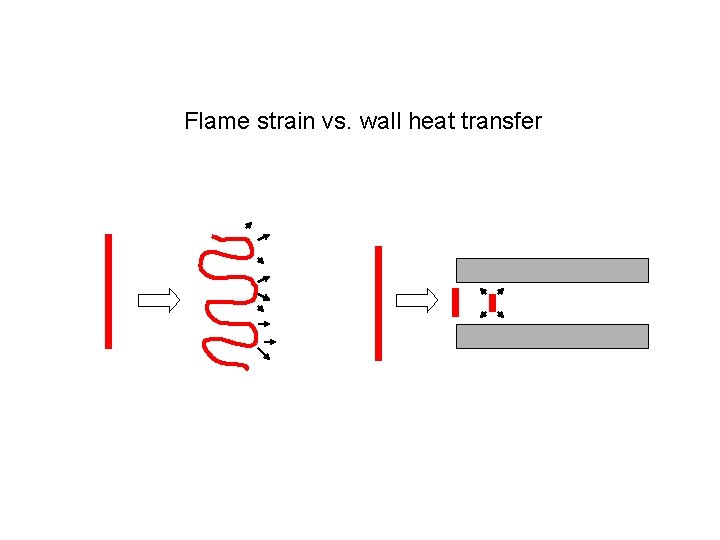
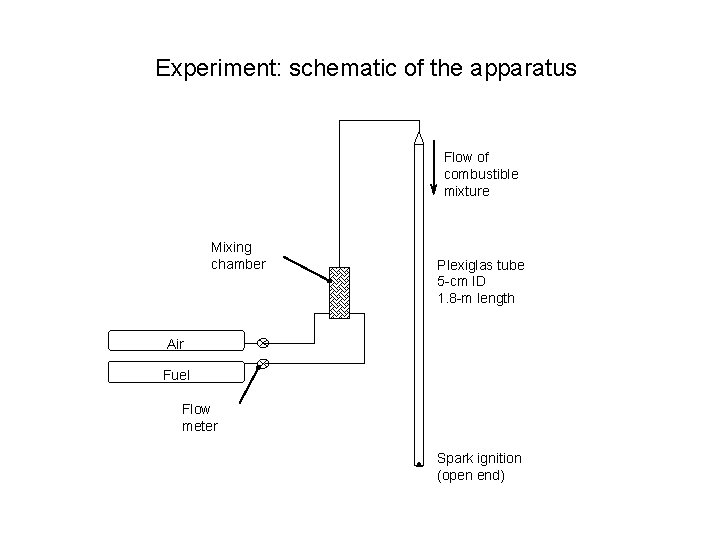
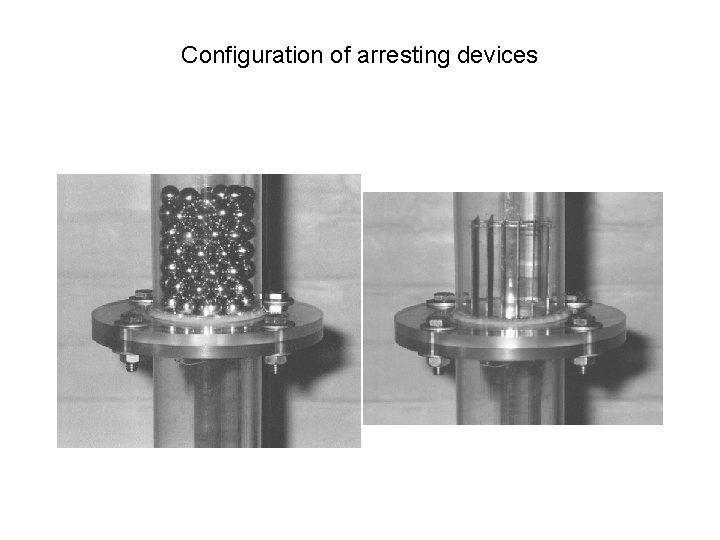
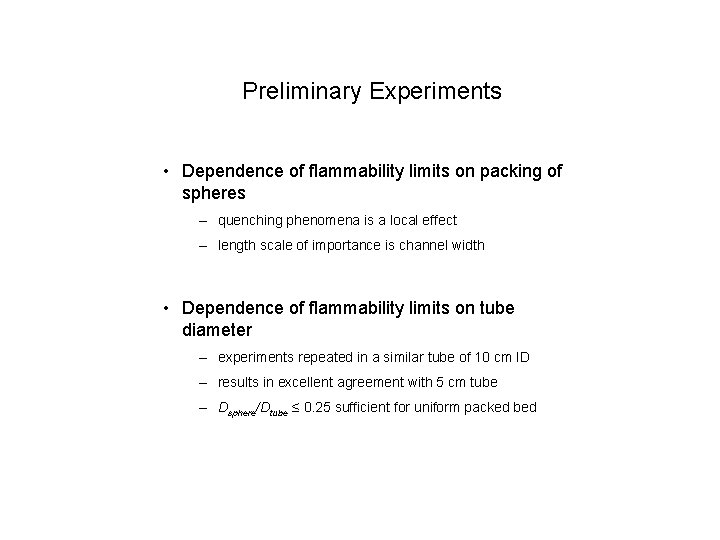
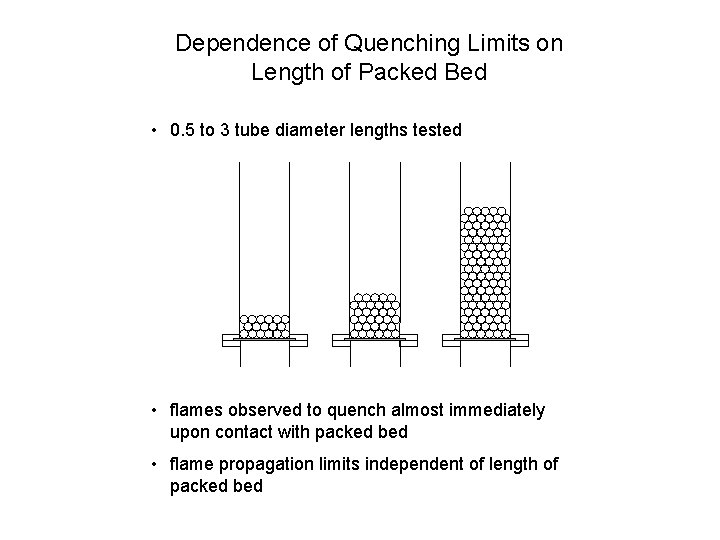
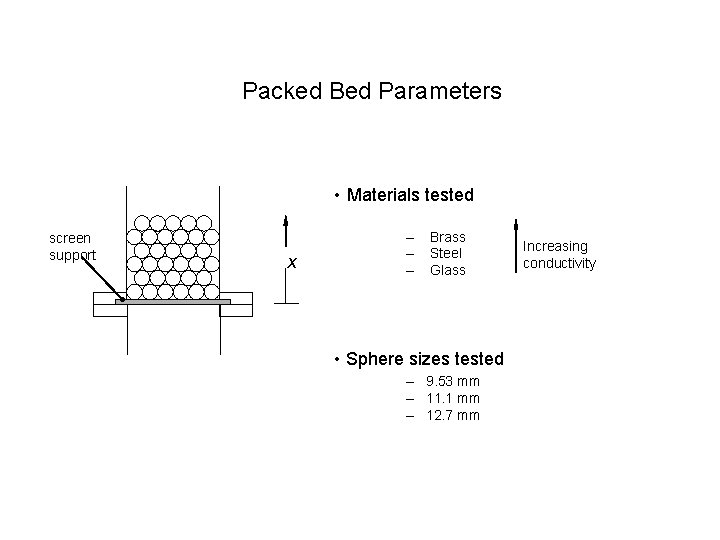
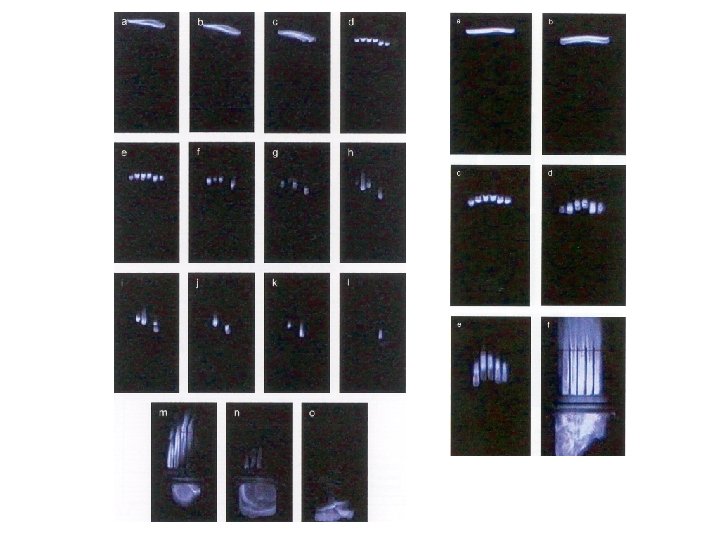
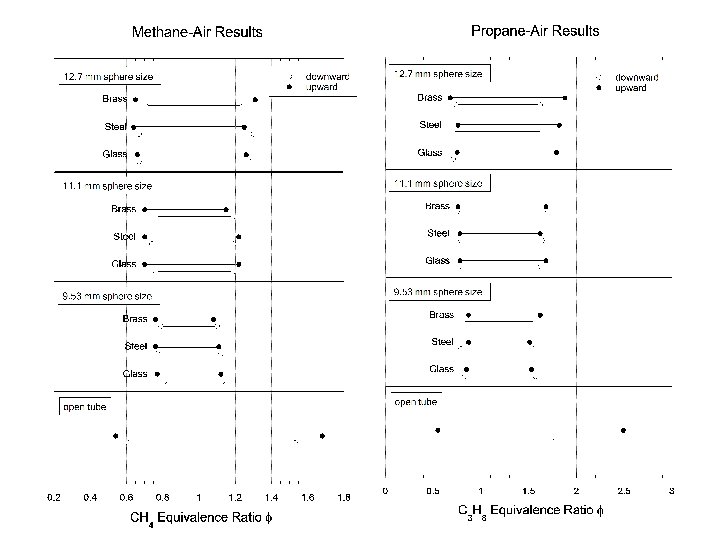
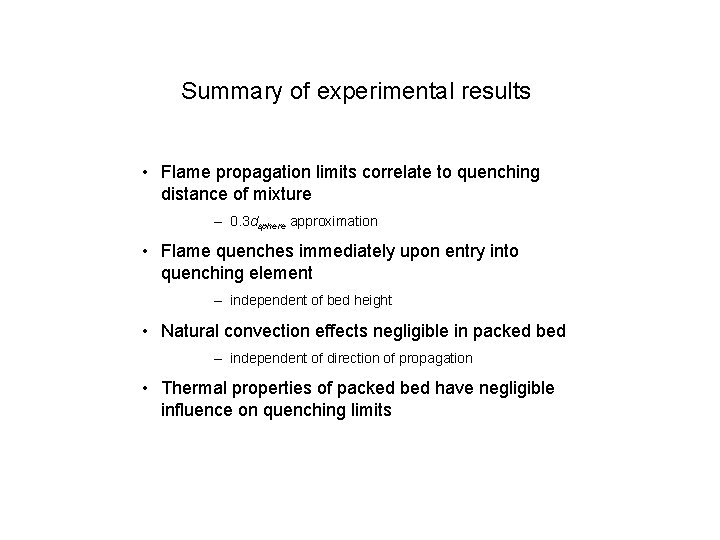
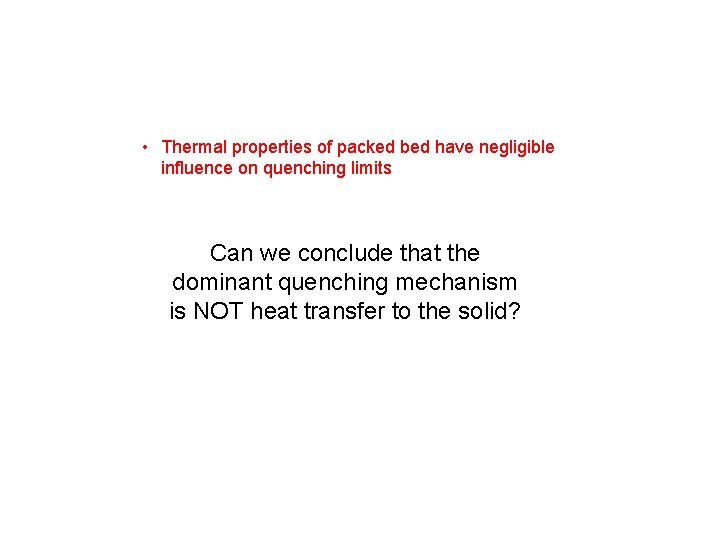
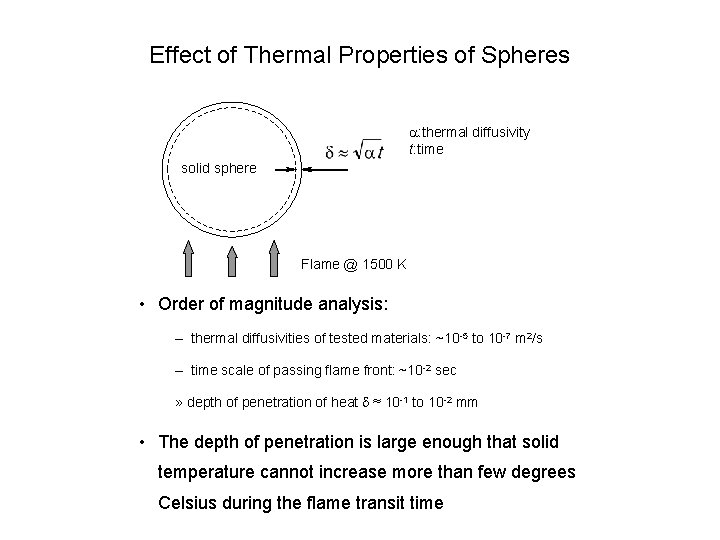
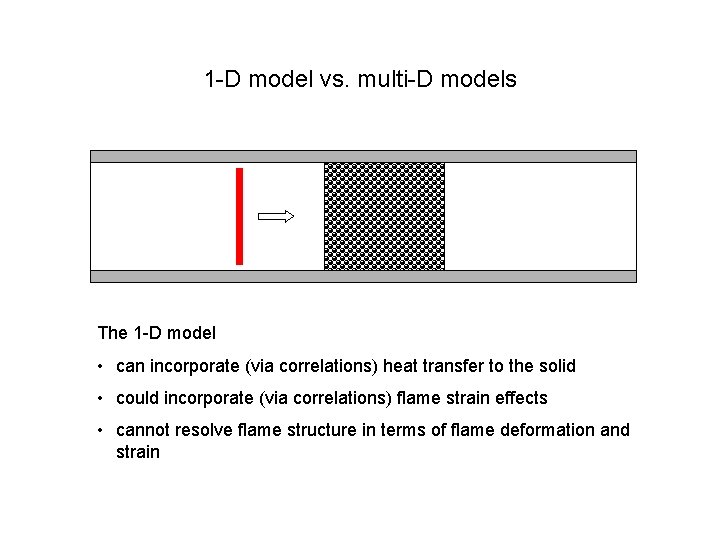
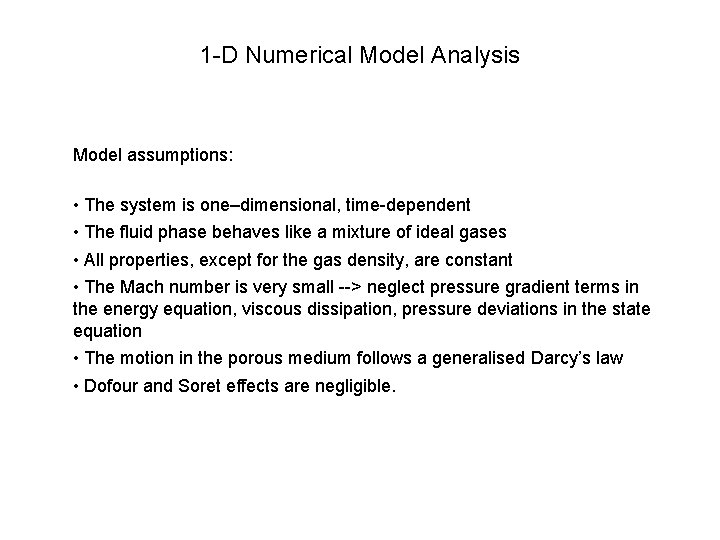
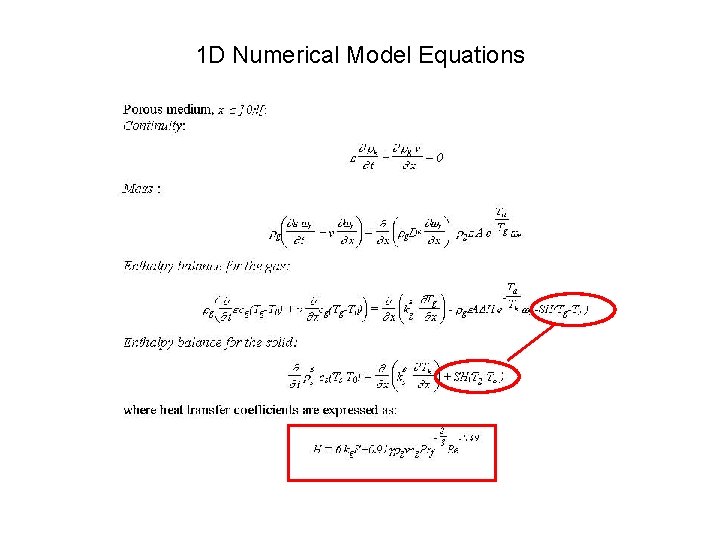
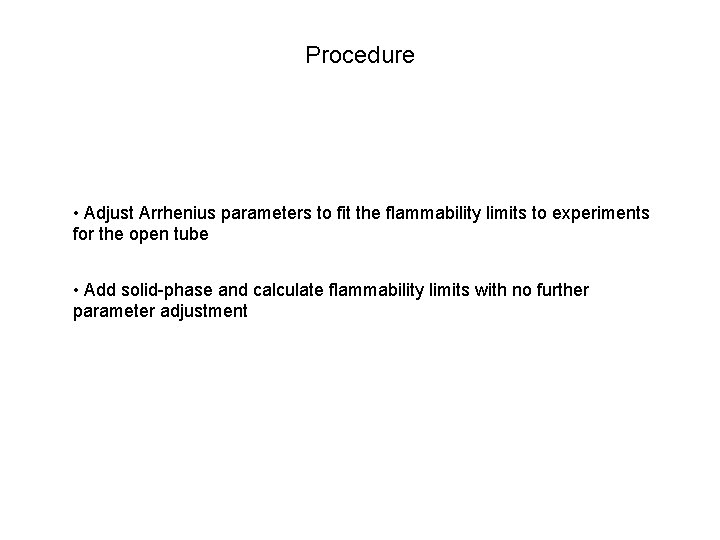
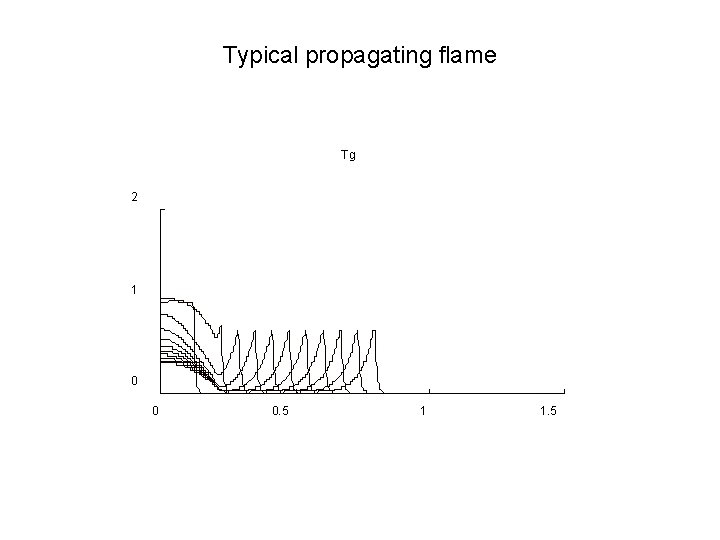
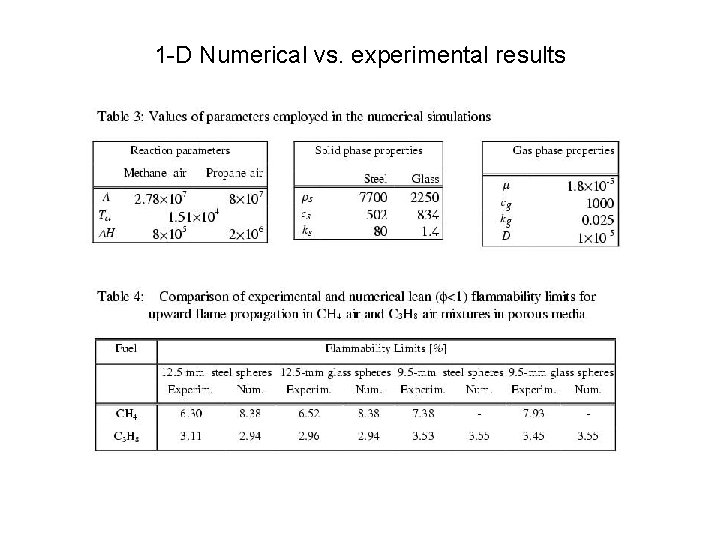
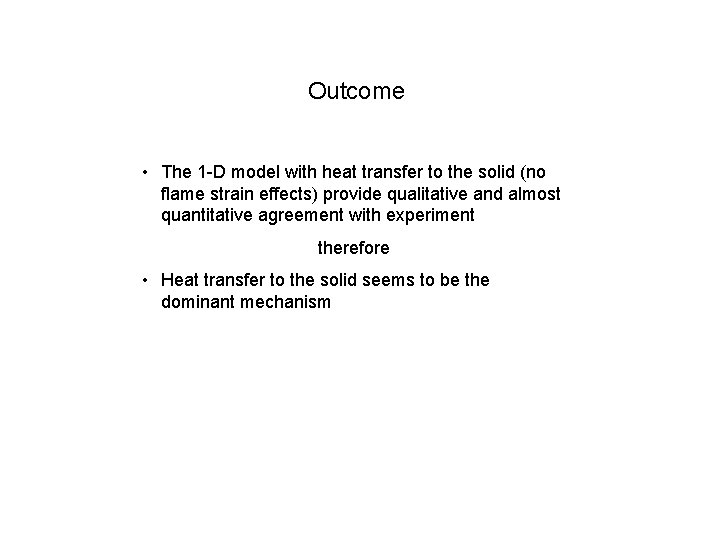
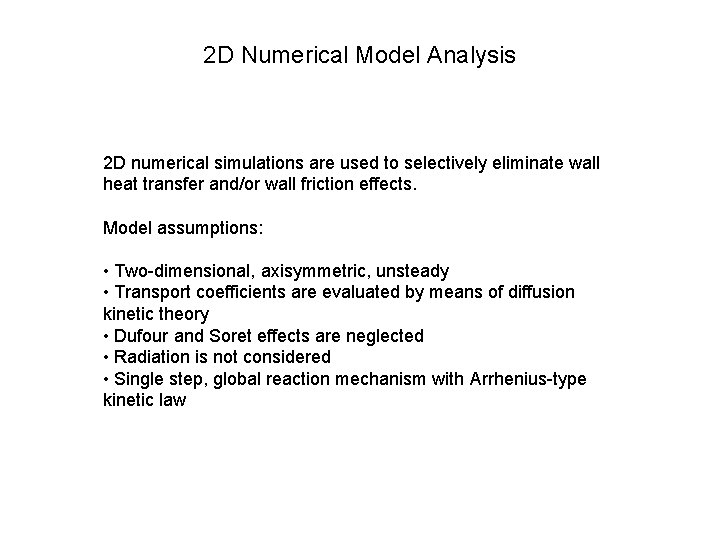
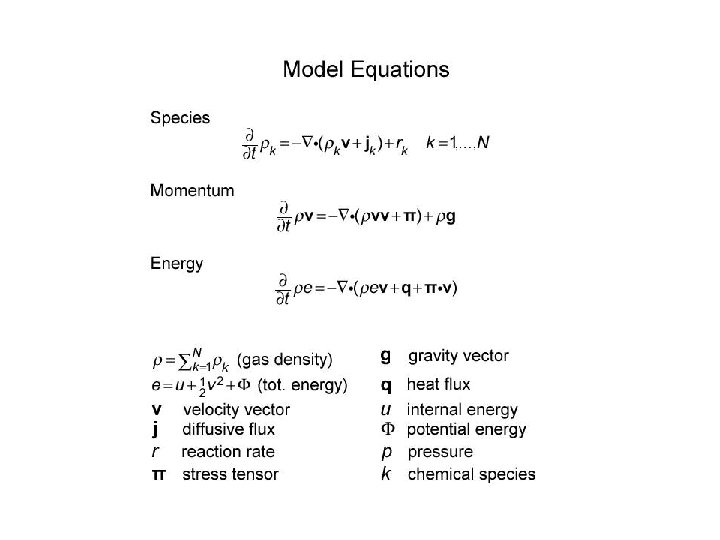
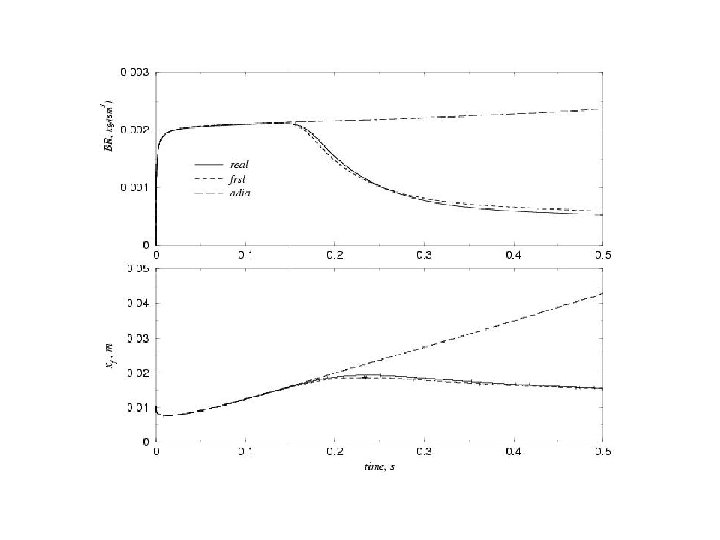
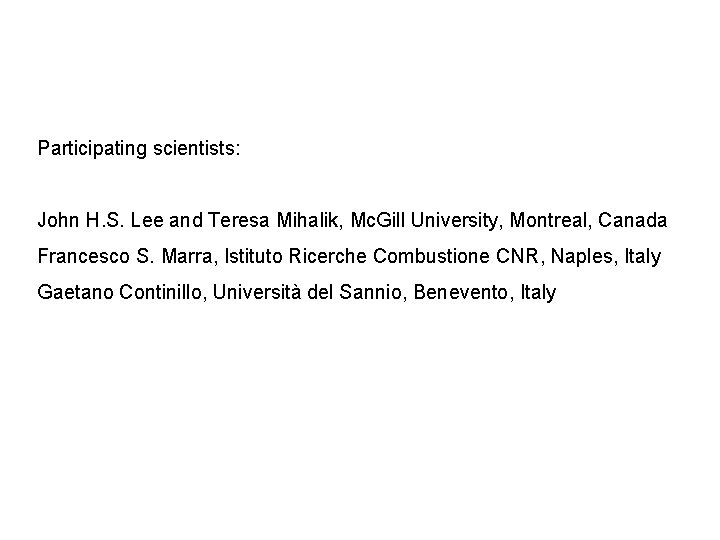
- Slides: 33
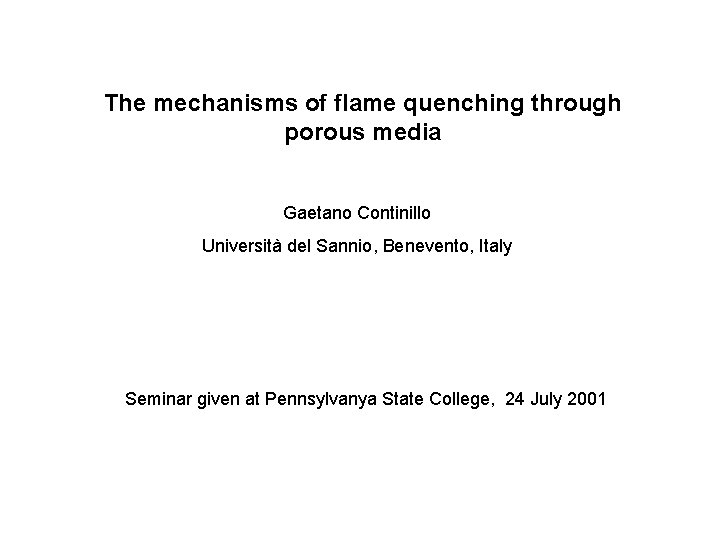
The mechanisms of flame quenching through porous media Gaetano Continillo Università del Sannio, Benevento, Italy Seminar given at Pennsylvanya State College, 24 July 2001
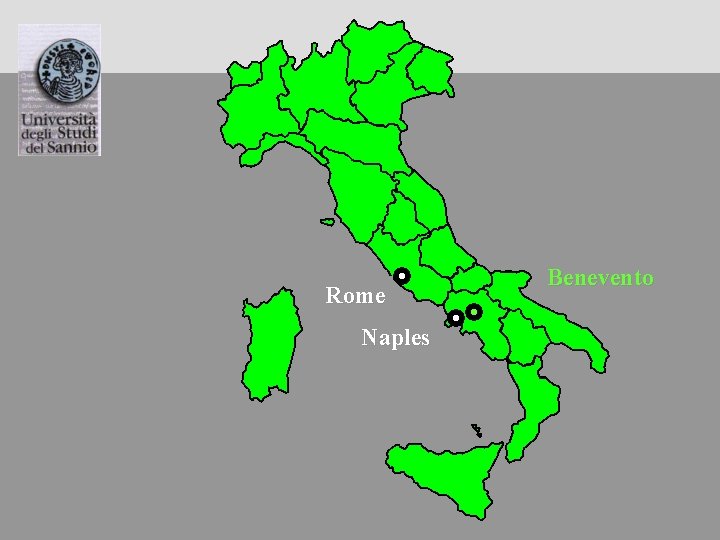
Rome Naples Benevento
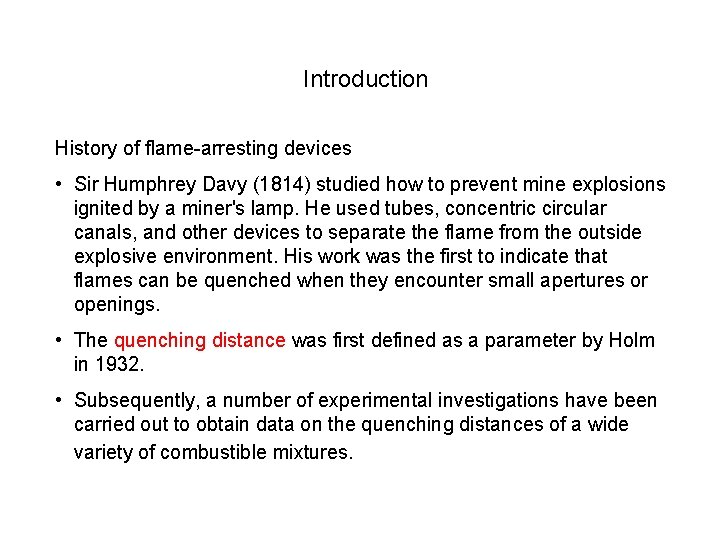
Introduction History of flame-arresting devices • Sir Humphrey Davy (1814) studied how to prevent mine explosions ignited by a miner's lamp. He used tubes, concentric circular canals, and other devices to separate the flame from the outside explosive environment. His work was the first to indicate that flames can be quenched when they encounter small apertures or openings. • The quenching distance was first defined as a parameter by Holm in 1932. • Subsequently, a number of experimental investigations have been carried out to obtain data on the quenching distances of a wide variety of combustible mixtures.
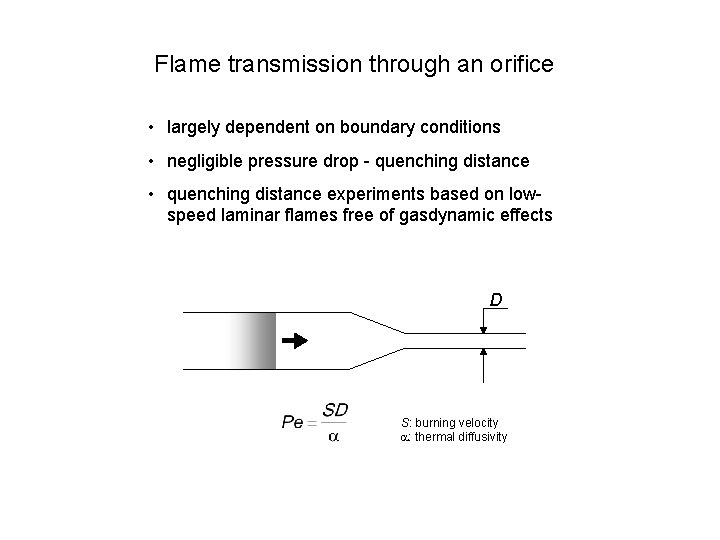
Flame transmission through an orifice • largely dependent on boundary conditions • negligible pressure drop - quenching distance • quenching distance experiments based on lowspeed laminar flames free of gasdynamic effects D S: burning velocity a: thermal diffusivity
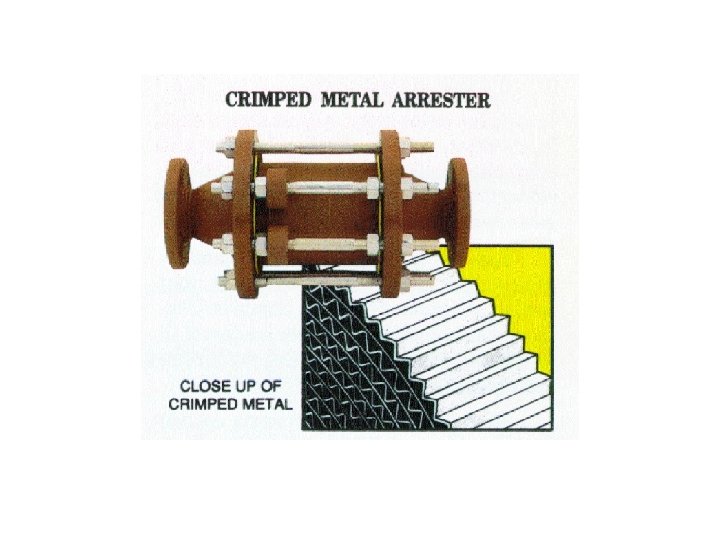
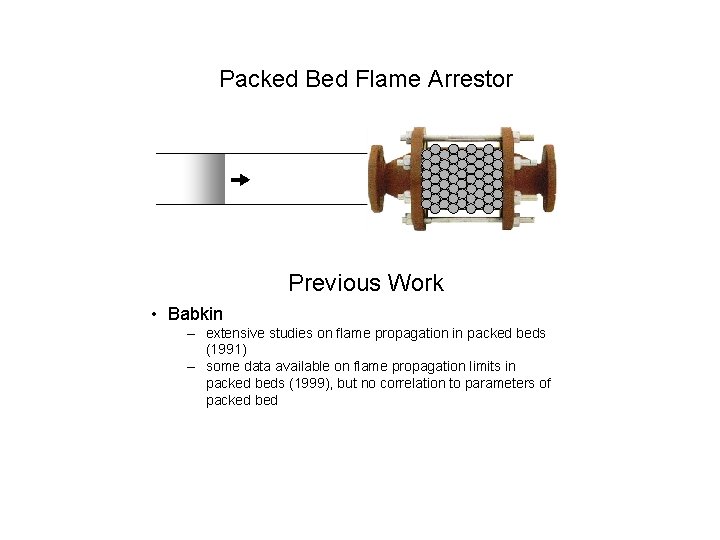
Packed Bed Flame Arrestor Previous Work • Babkin – extensive studies on flame propagation in packed beds (1991) – some data available on flame propagation limits in packed beds (1999), but no correlation to parameters of packed bed
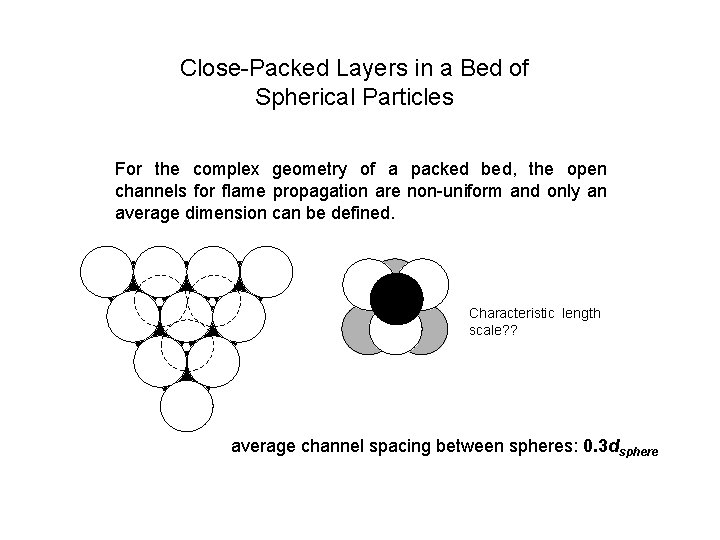
Close-Packed Layers in a Bed of Spherical Particles For the complex geometry of a packed bed, the open channels for flame propagation are non-uniform and only an average dimension can be defined. Characteristic length scale? ? average channel spacing between spheres: 0. 3 dsphere
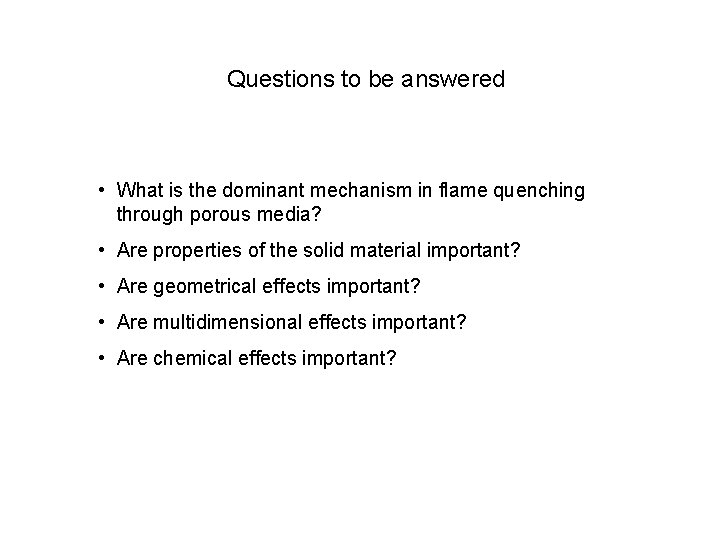
Questions to be answered • What is the dominant mechanism in flame quenching through porous media? • Are properties of the solid material important? • Are geometrical effects important? • Are multidimensional effects important? • Are chemical effects important?
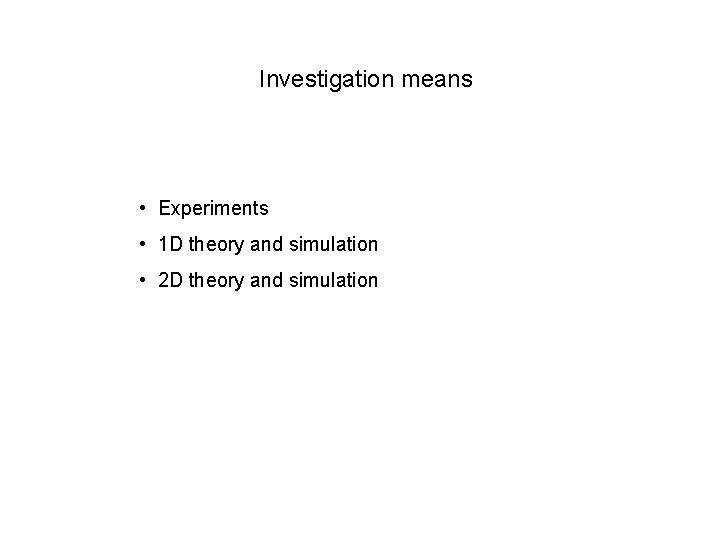
Investigation means • Experiments • 1 D theory and simulation • 2 D theory and simulation
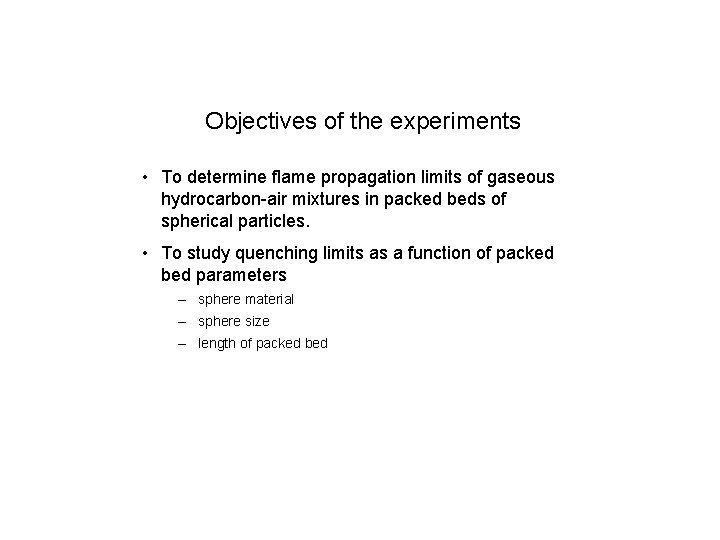
Objectives of the experiments • To determine flame propagation limits of gaseous hydrocarbon-air mixtures in packed beds of spherical particles. • To study quenching limits as a function of packed bed parameters – sphere material – sphere size – length of packed bed
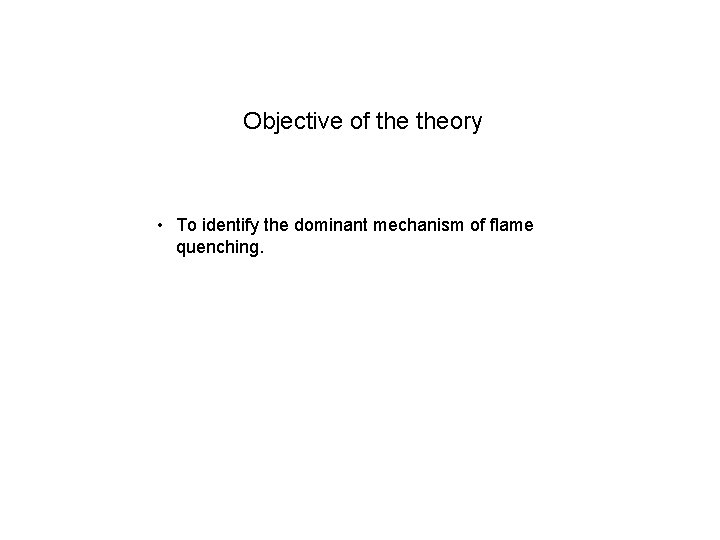
Objective of theory • To identify the dominant mechanism of flame quenching.
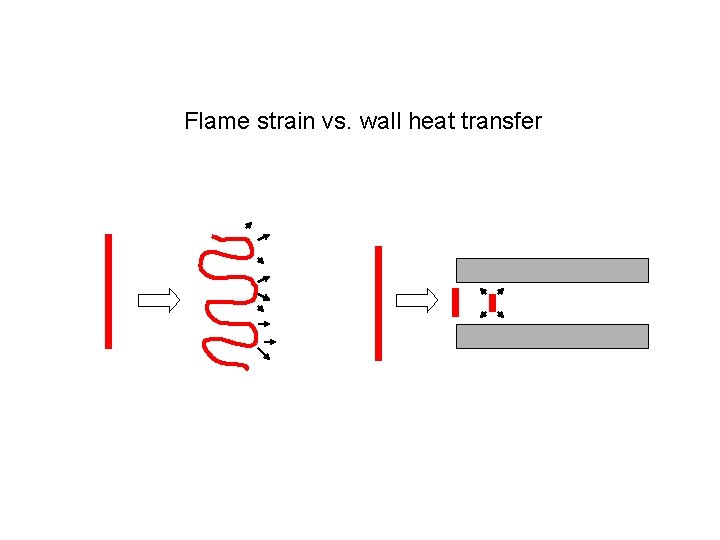
Flame strain vs. wall heat transfer
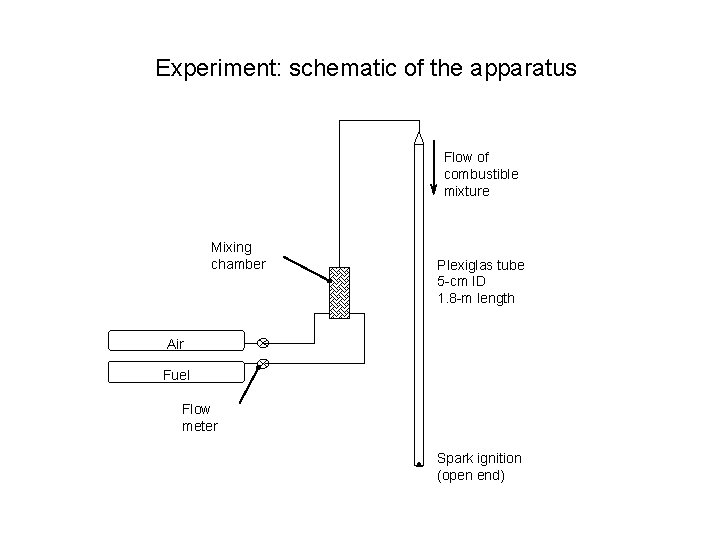
Experiment: schematic of the apparatus Flow of combustible mixture Mixing chamber Plexiglas tube 5 -cm ID 1. 8 -m length Air Fuel Flow meter Spark ignition (open end)
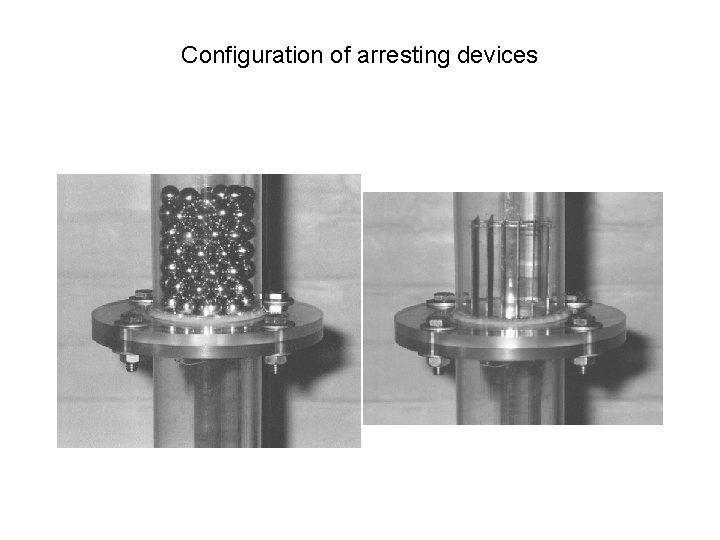
Configuration of arresting devices
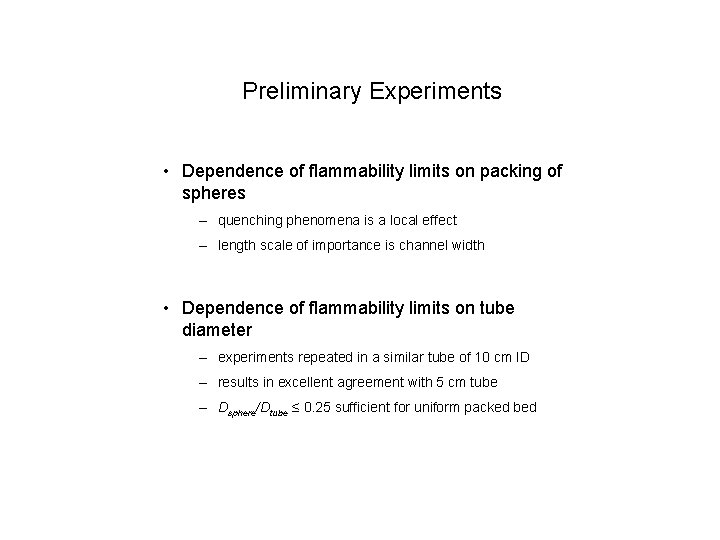
Preliminary Experiments • Dependence of flammability limits on packing of spheres – quenching phenomena is a local effect – length scale of importance is channel width • Dependence of flammability limits on tube diameter – experiments repeated in a similar tube of 10 cm ID – results in excellent agreement with 5 cm tube – Dsphere/Dtube ≤ 0. 25 sufficient for uniform packed bed
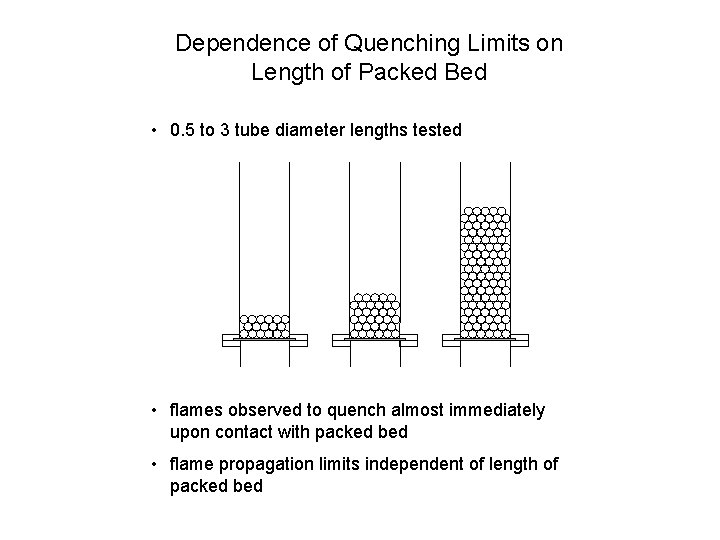
Dependence of Quenching Limits on Length of Packed Bed • 0. 5 to 3 tube diameter lengths tested • flames observed to quench almost immediately upon contact with packed bed • flame propagation limits independent of length of packed bed
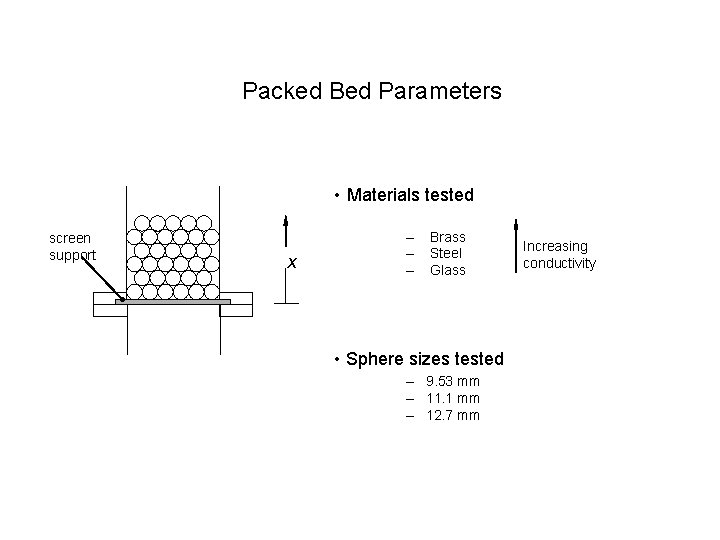
Packed Bed Parameters • Materials tested screen support x – – – Brass Steel Glass • Sphere sizes tested – 9. 53 mm – 11. 1 mm – 12. 7 mm Increasing conductivity
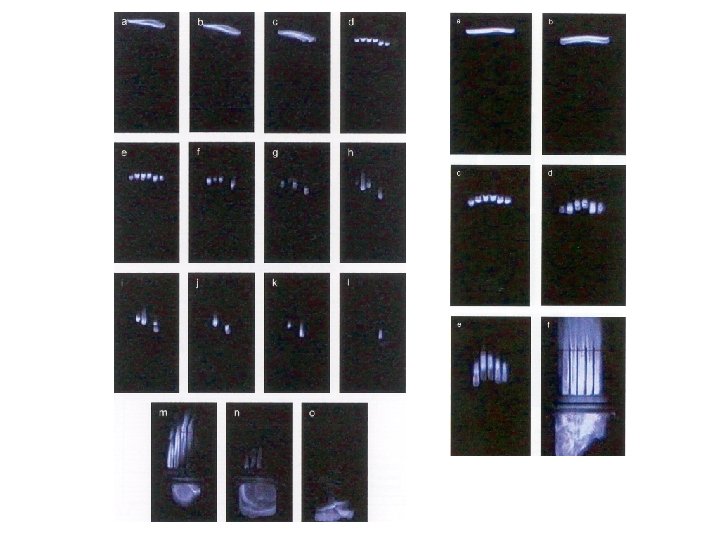
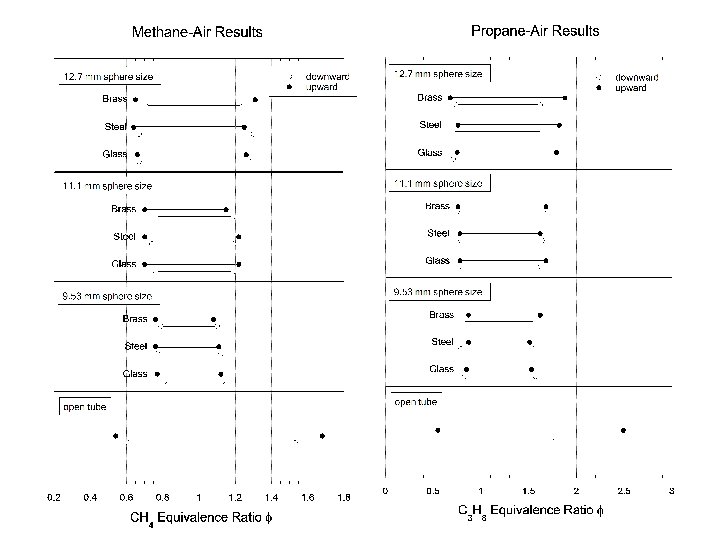
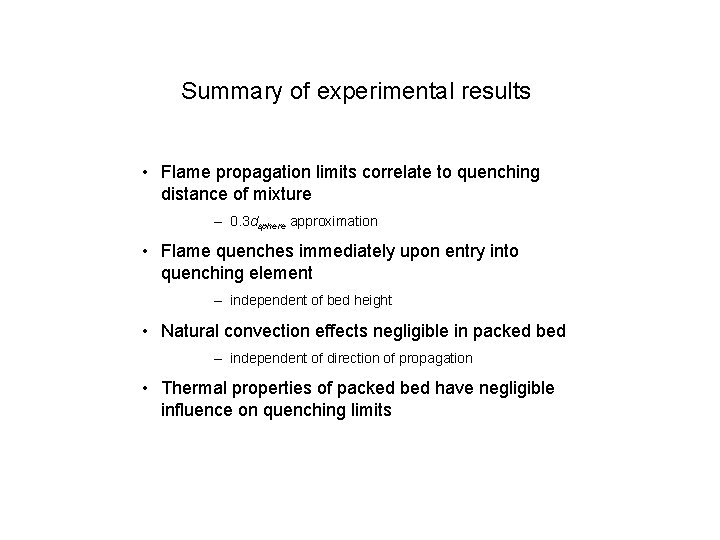
Summary of experimental results • Flame propagation limits correlate to quenching distance of mixture – 0. 3 dsphere approximation • Flame quenches immediately upon entry into quenching element – independent of bed height • Natural convection effects negligible in packed bed – independent of direction of propagation • Thermal properties of packed bed have negligible influence on quenching limits
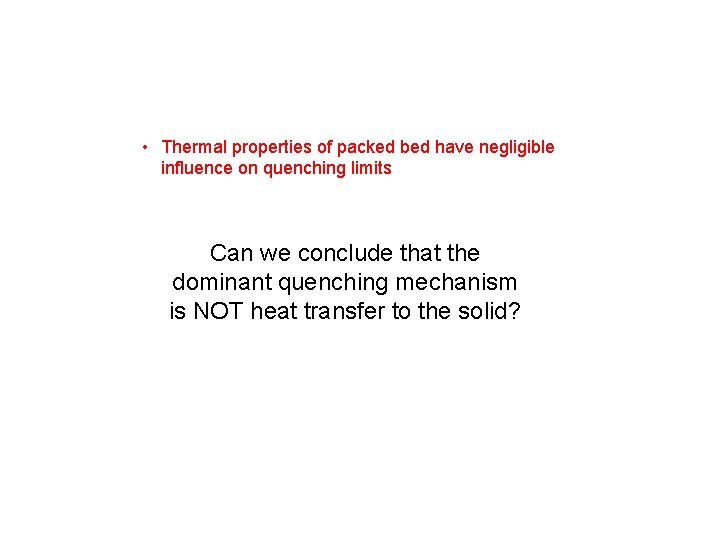
• Thermal properties of packed bed have negligible influence on quenching limits Can we conclude that the dominant quenching mechanism is NOT heat transfer to the solid?
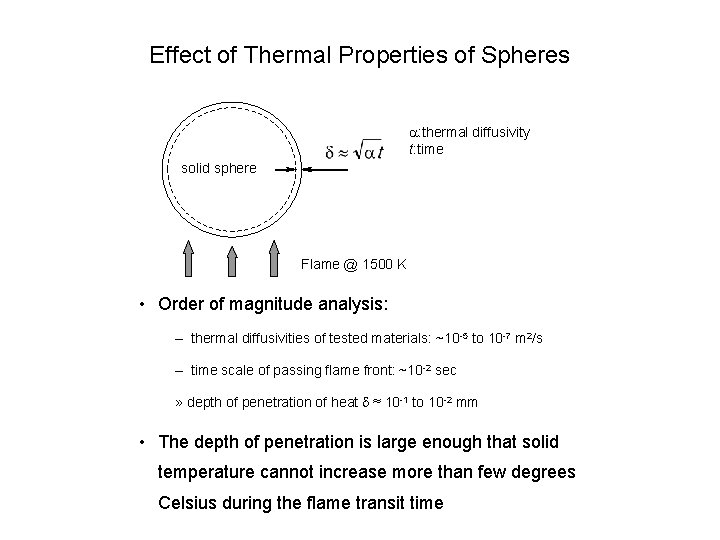
Effect of Thermal Properties of Spheres a: thermal diffusivity t: time solid sphere Flame @ 1500 K • Order of magnitude analysis: – thermal diffusivities of tested materials: ~10 -5 to 10 -7 m 2/s – time scale of passing flame front: ~10 -2 sec » depth of penetration of heat d ≈ 10 -1 to 10 -2 mm • The depth of penetration is large enough that solid temperature cannot increase more than few degrees Celsius during the flame transit time
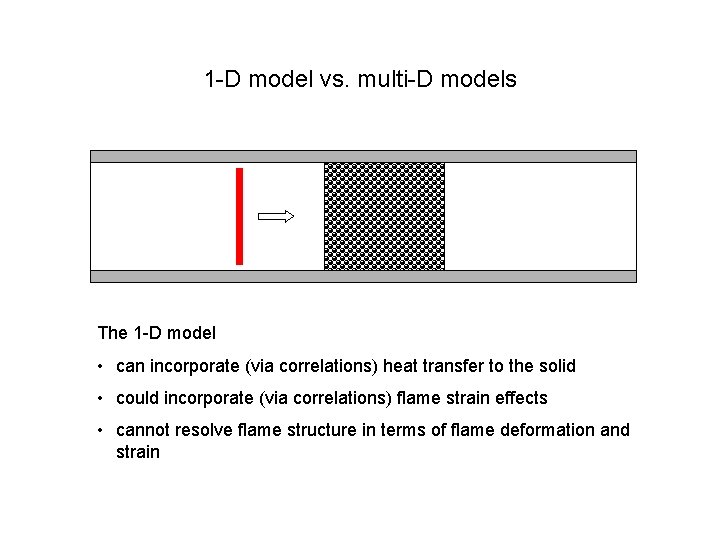
1 -D model vs. multi-D models The 1 -D model • can incorporate (via correlations) heat transfer to the solid • could incorporate (via correlations) flame strain effects • cannot resolve flame structure in terms of flame deformation and strain
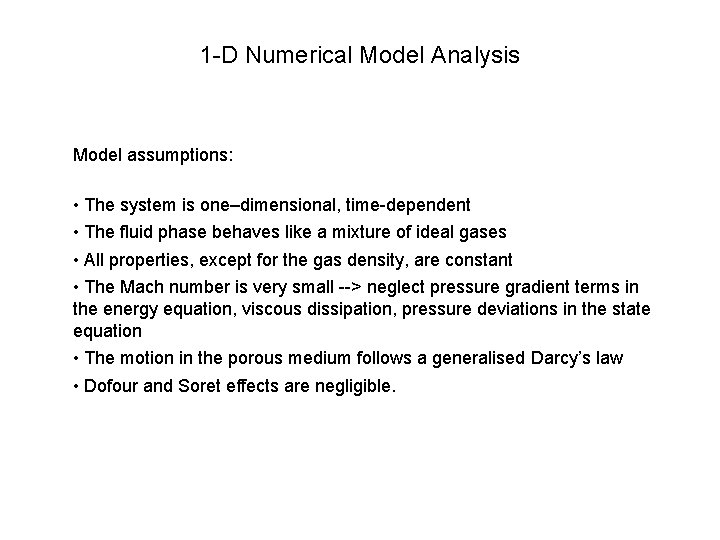
1 -D Numerical Model Analysis Model assumptions: • The system is one–dimensional, time-dependent • The fluid phase behaves like a mixture of ideal gases • All properties, except for the gas density, are constant • The Mach number is very small --> neglect pressure gradient terms in the energy equation, viscous dissipation, pressure deviations in the state equation • The motion in the porous medium follows a generalised Darcy’s law • Dofour and Soret effects are negligible.
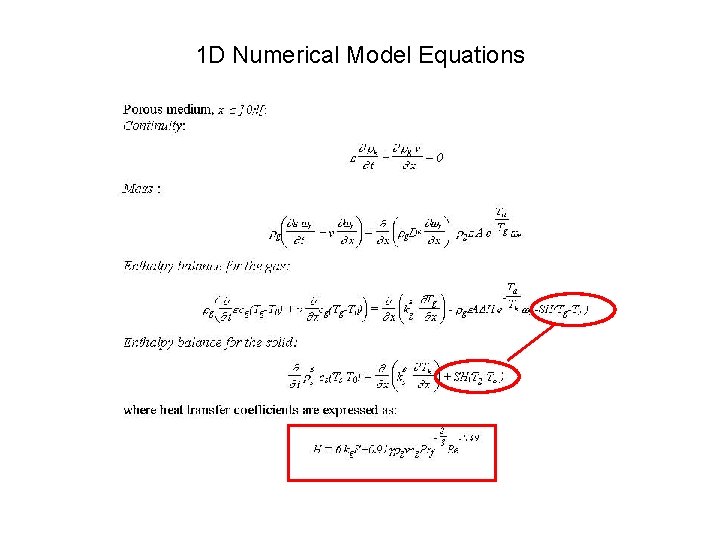
1 D Numerical Model Equations
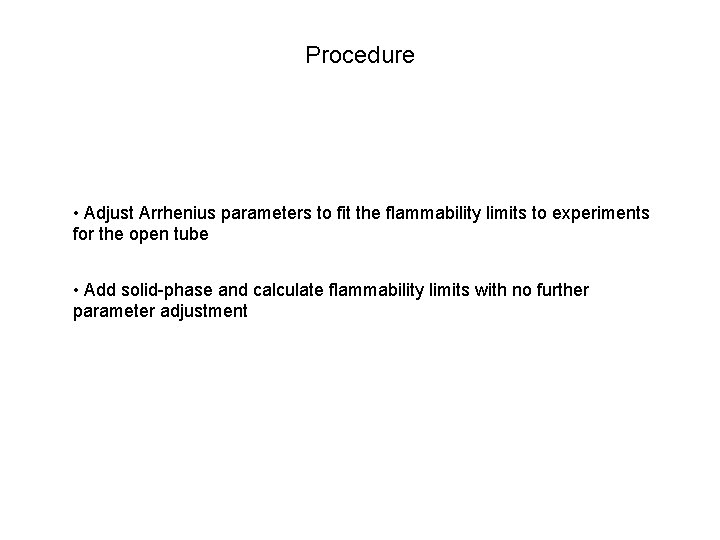
Procedure • Adjust Arrhenius parameters to fit the flammability limits to experiments for the open tube • Add solid-phase and calculate flammability limits with no further parameter adjustment
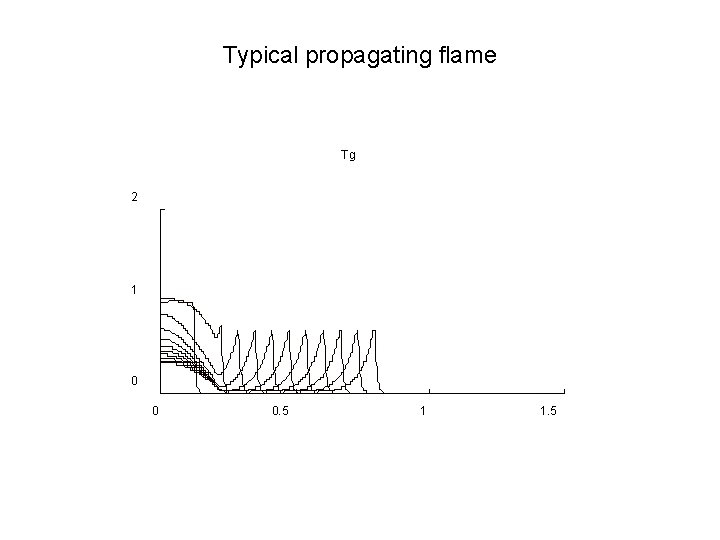
Typical propagating flame Tg 2 1 0 0 0. 5 1 1. 5
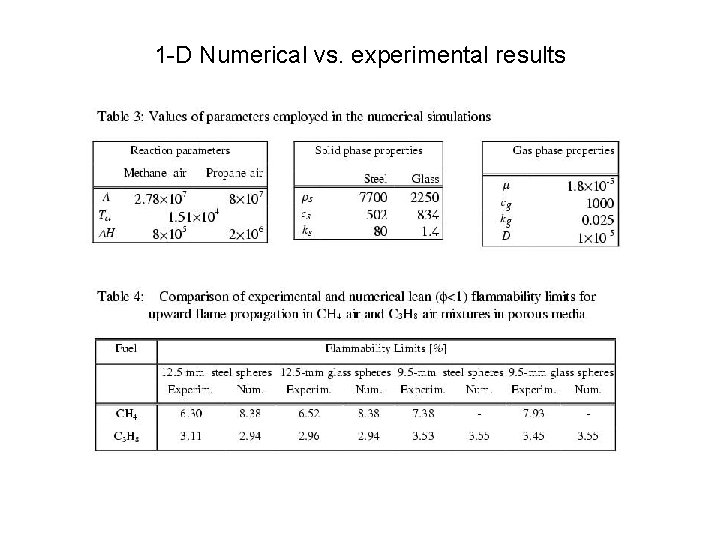
1 -D Numerical vs. experimental results
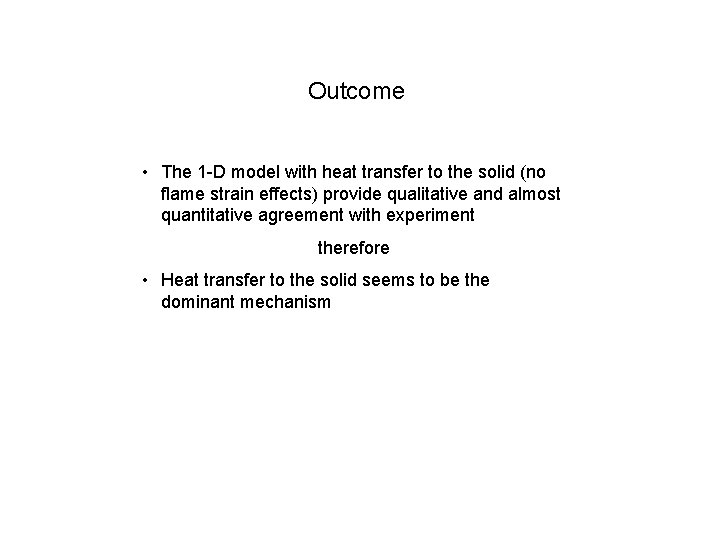
Outcome • The 1 -D model with heat transfer to the solid (no flame strain effects) provide qualitative and almost quantitative agreement with experiment therefore • Heat transfer to the solid seems to be the dominant mechanism
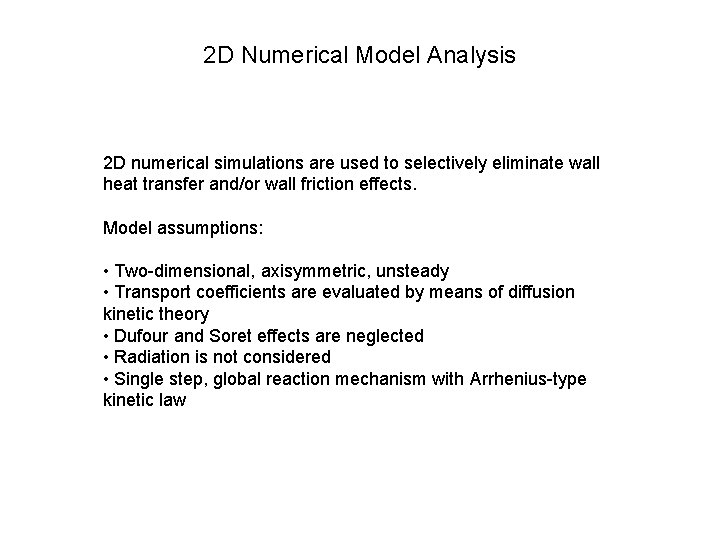
2 D Numerical Model Analysis 2 D numerical simulations are used to selectively eliminate wall heat transfer and/or wall friction effects. Model assumptions: • Two-dimensional, axisymmetric, unsteady • Transport coefficients are evaluated by means of diffusion kinetic theory • Dufour and Soret effects are neglected • Radiation is not considered • Single step, global reaction mechanism with Arrhenius-type kinetic law
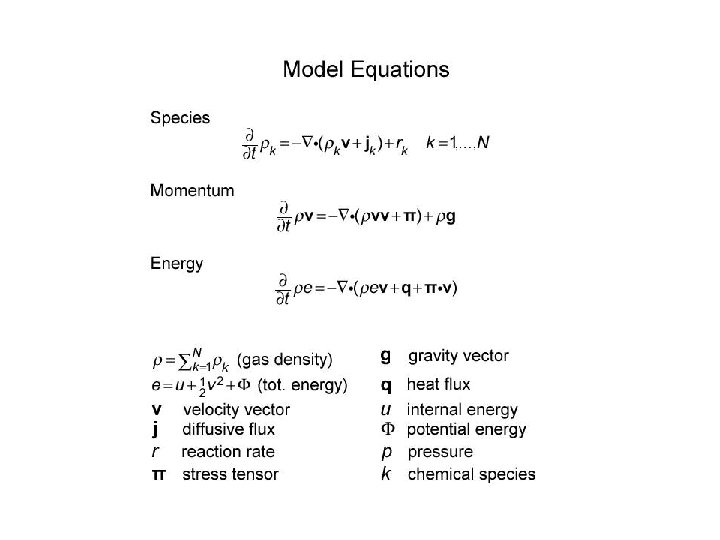
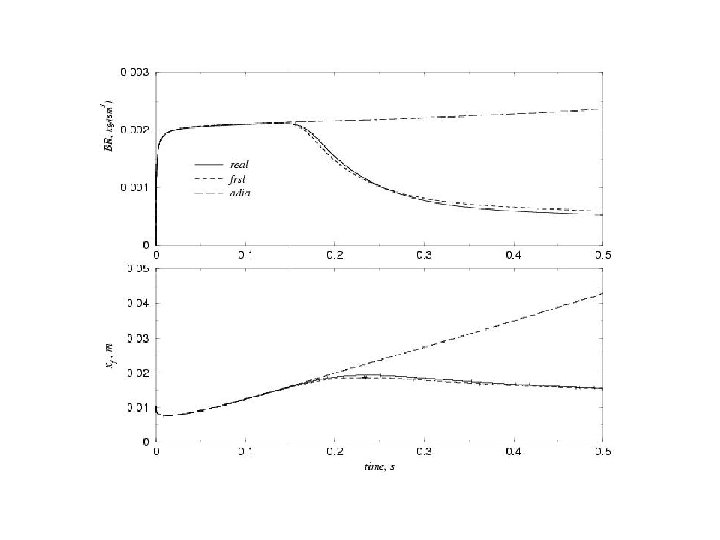
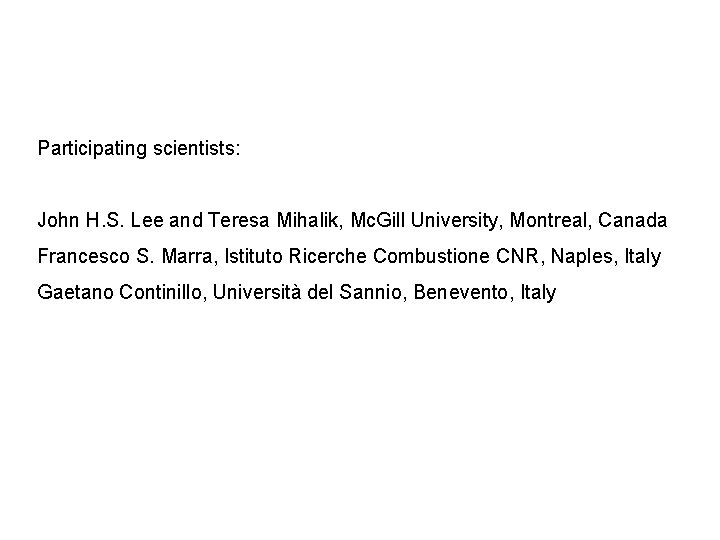
Participating scientists: John H. S. Lee and Teresa Mihalik, Mc. Gill University, Montreal, Canada Francesco S. Marra, Istituto Ricerche Combustione CNR, Naples, Italy Gaetano Continillo, Università del Sannio, Benevento, Italy
Quenching
Heat transfer in porous media comsol
List of symbols
Why is the yellow flame called the safety flame
Types of porous absorbers
Geotekstil separator kelas 1
Porous welds
Porous material
Shaud tavakoli
Bahan drainase porous atau penyaring (filter)
By one man sin
Classes of furcation
Tangential sawing of timber
The poem night of the scorpion
Hình ảnh bộ gõ cơ thể búng tay
Slidetodoc
Bổ thể
Tỉ lệ cơ thể trẻ em
Chó sói
Thang điểm glasgow
Chúa yêu trần thế alleluia
Môn thể thao bắt đầu bằng từ chạy
Thế nào là hệ số cao nhất
Các châu lục và đại dương trên thế giới
Công thức tính độ biến thiên đông lượng
Trời xanh đây là của chúng ta thể thơ
Mật thư anh em như thể tay chân
Làm thế nào để 102-1=99
độ dài liên kết
Các châu lục và đại dương trên thế giới
Thơ thất ngôn tứ tuyệt đường luật
Quá trình desamine hóa có thể tạo ra
Một số thể thơ truyền thống
Cái miệng nó xinh thế chỉ nói điều hay thôi