TECHNOLOGIES FOR PARTICULATE EMISSION CONTROL TECHNOLOGIES FOR PARTICULATE
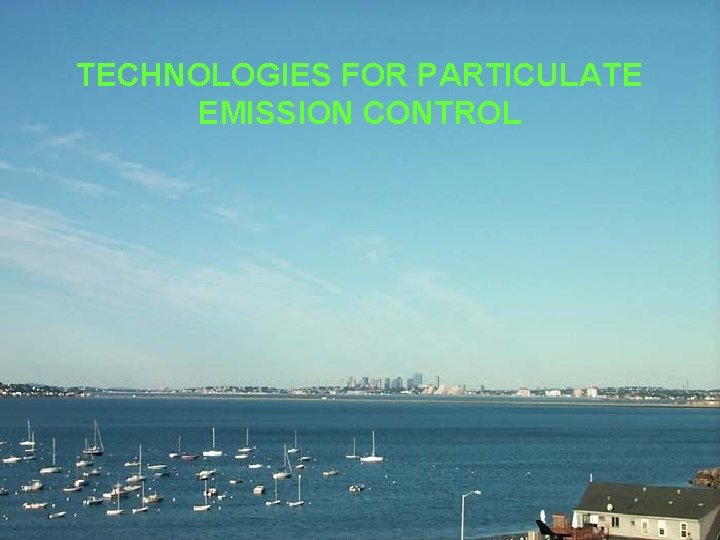
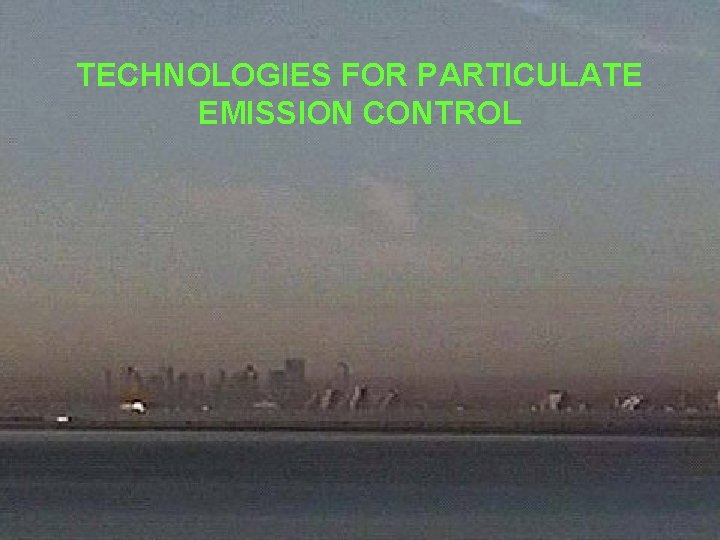
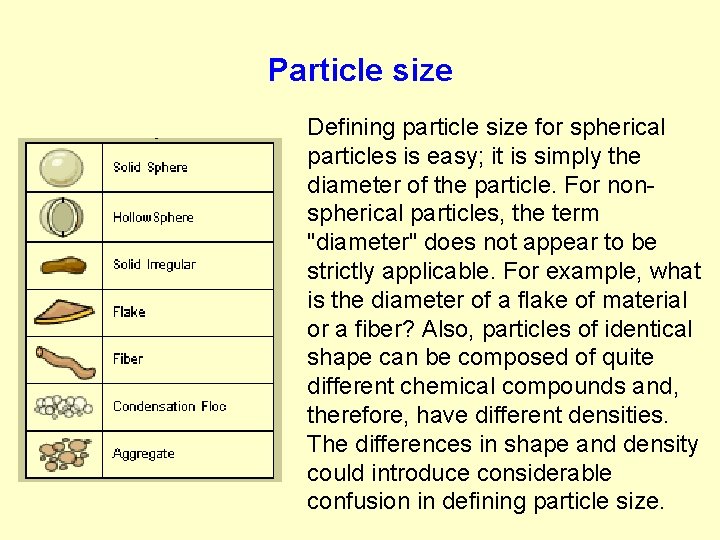
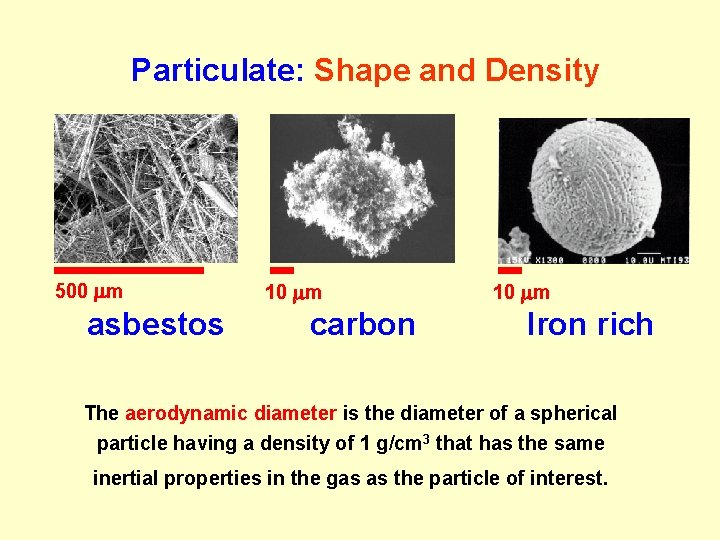
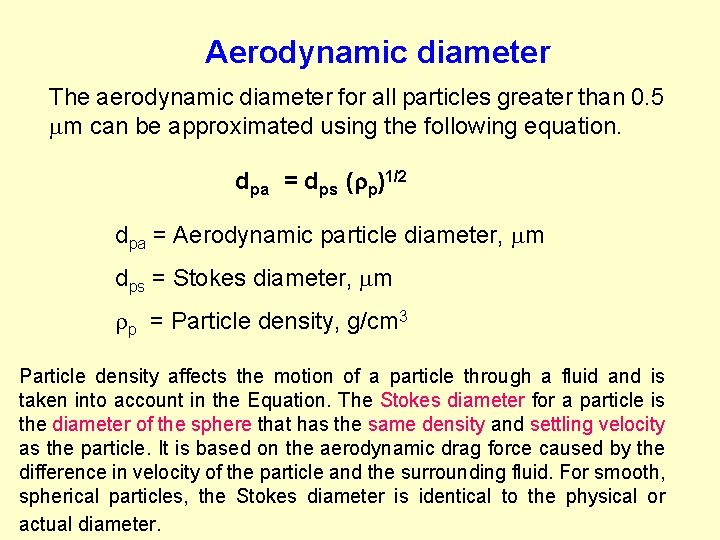
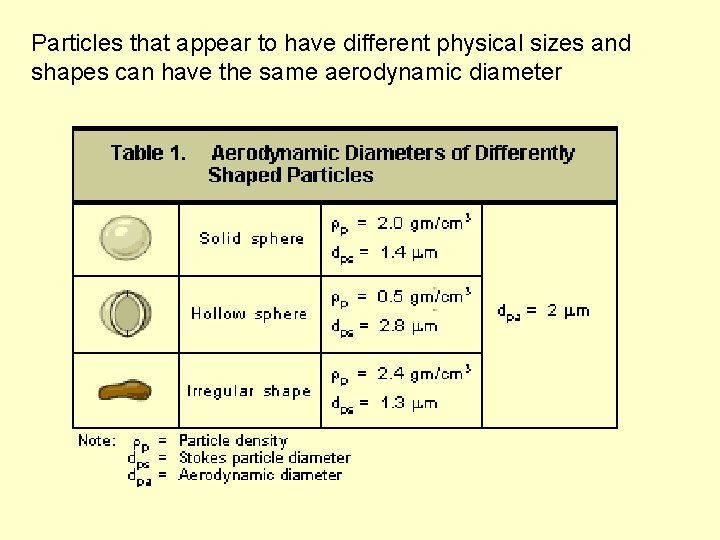
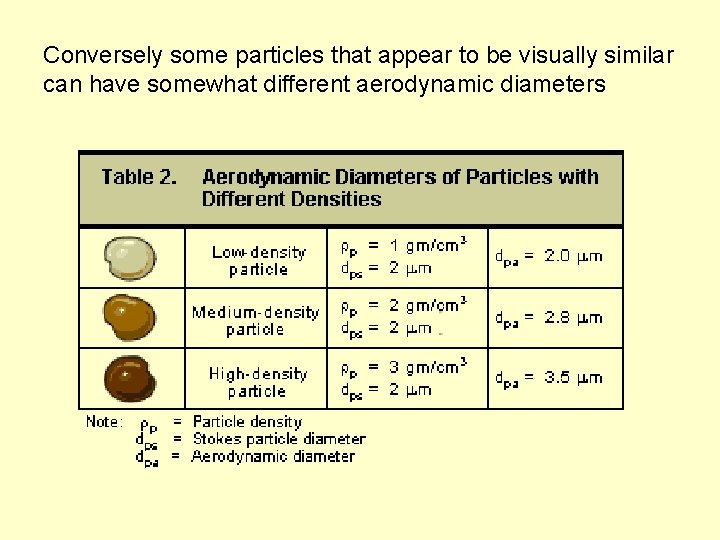
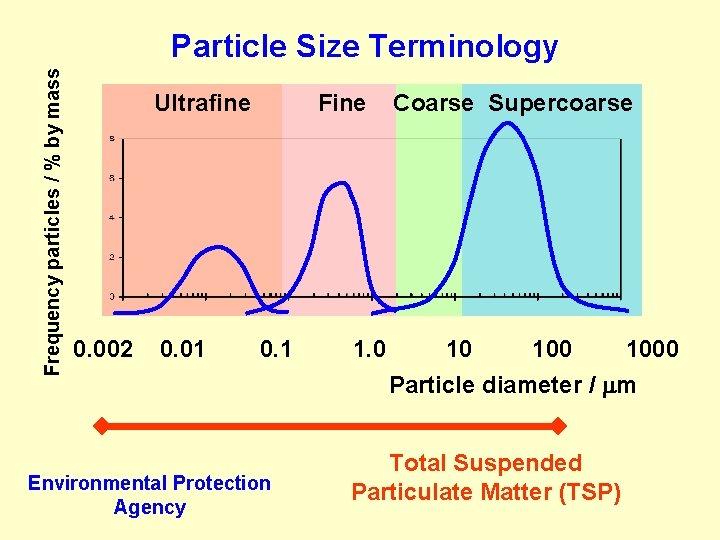
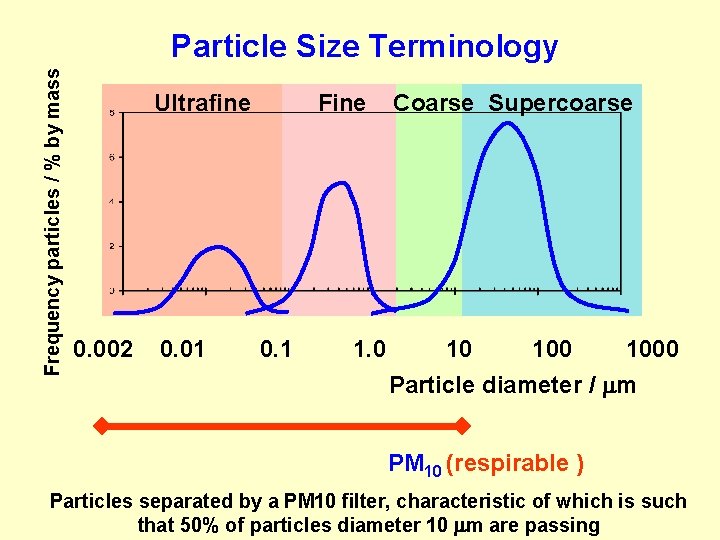
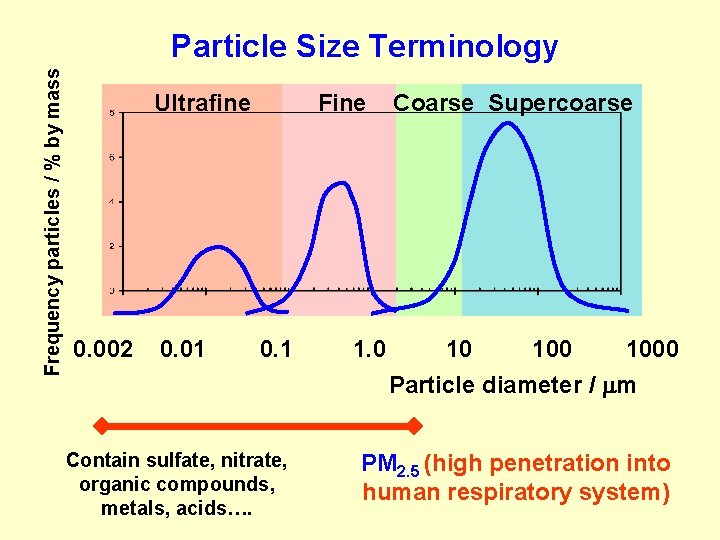
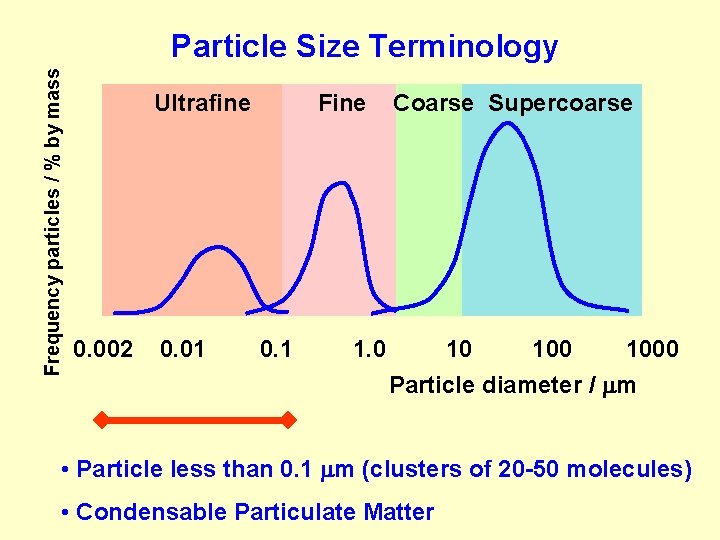
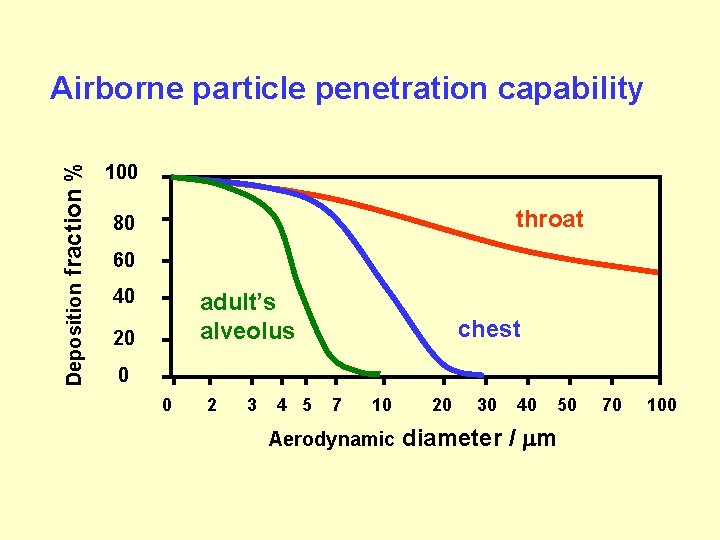
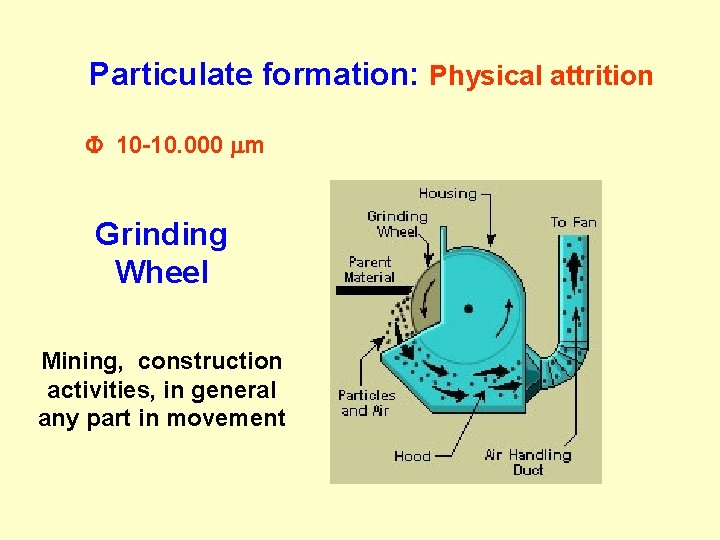
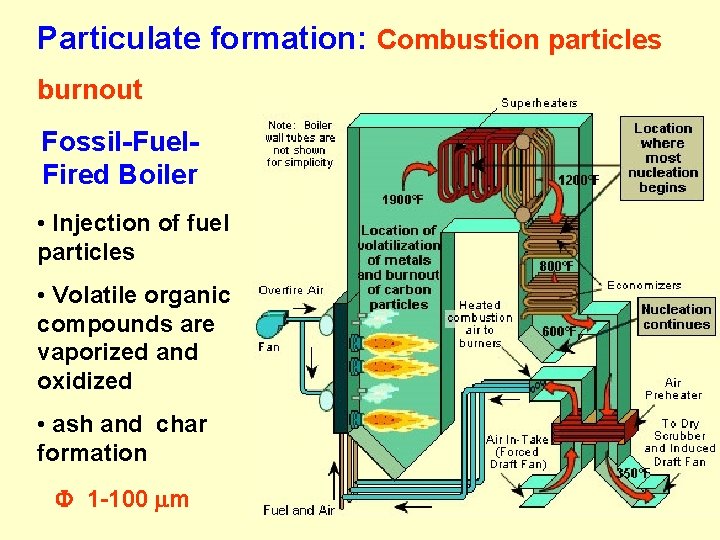
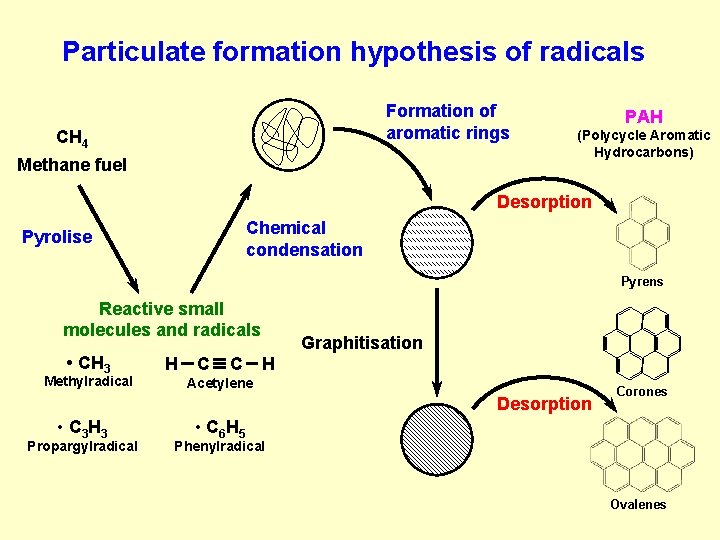
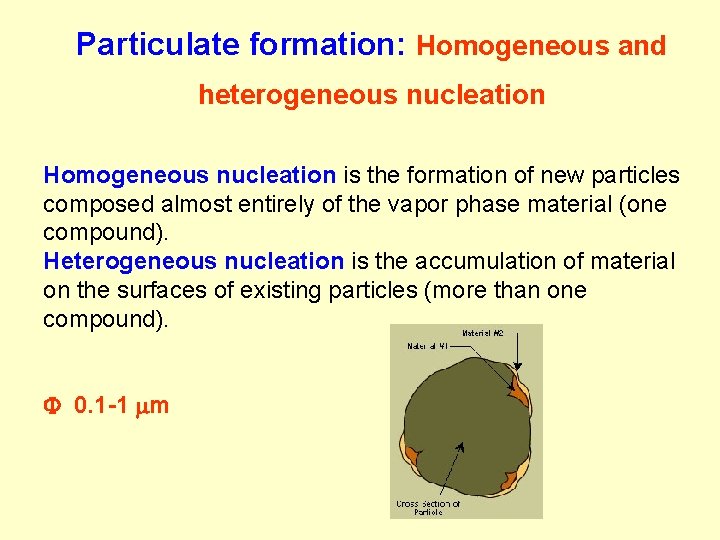
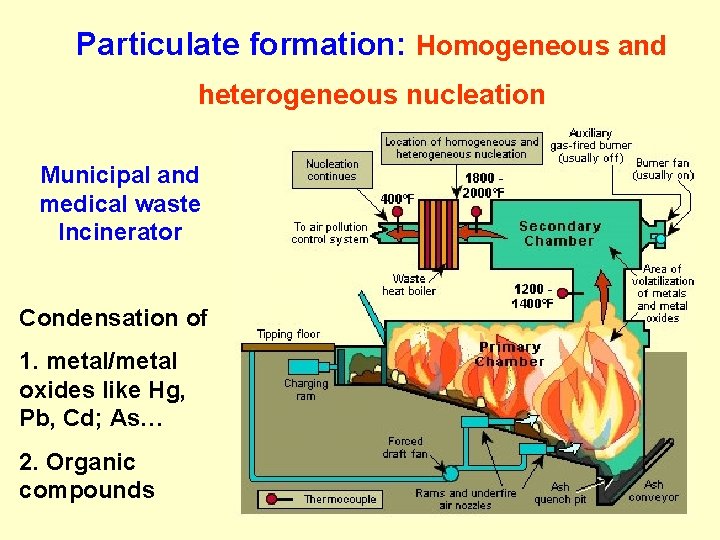
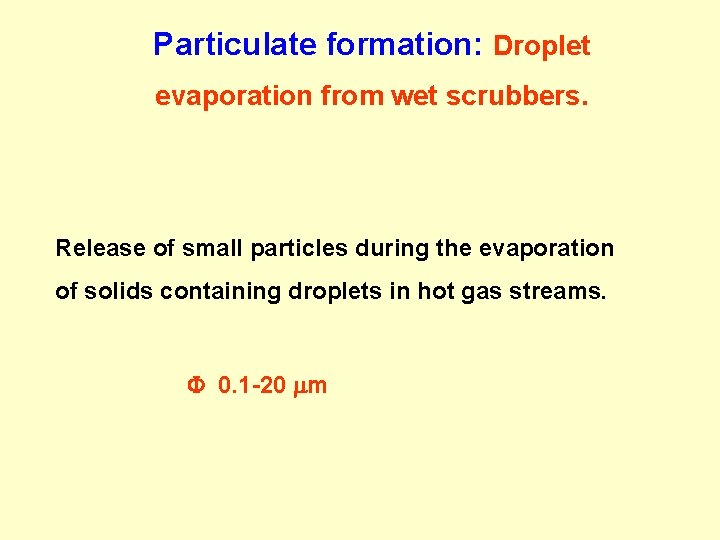
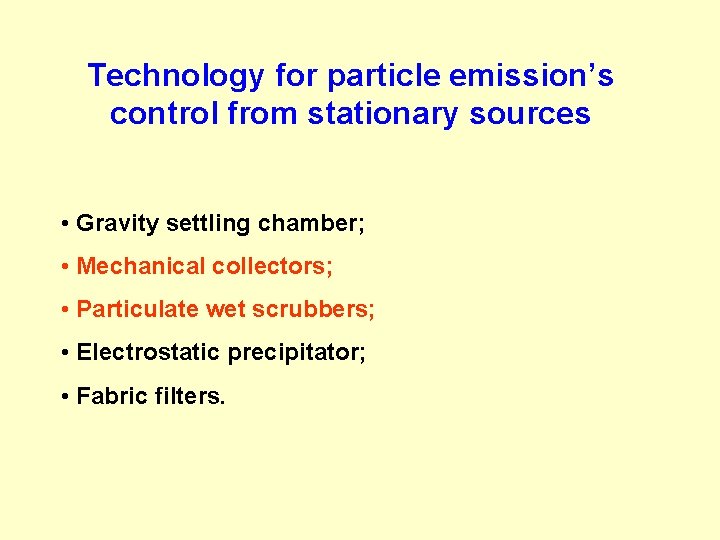
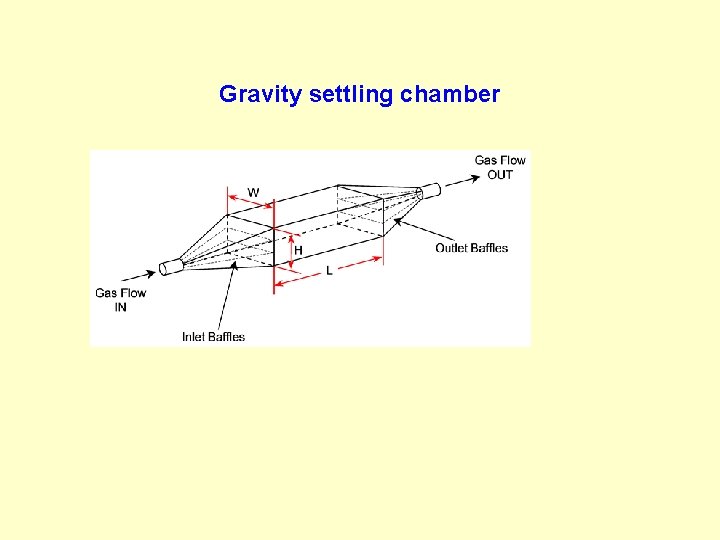
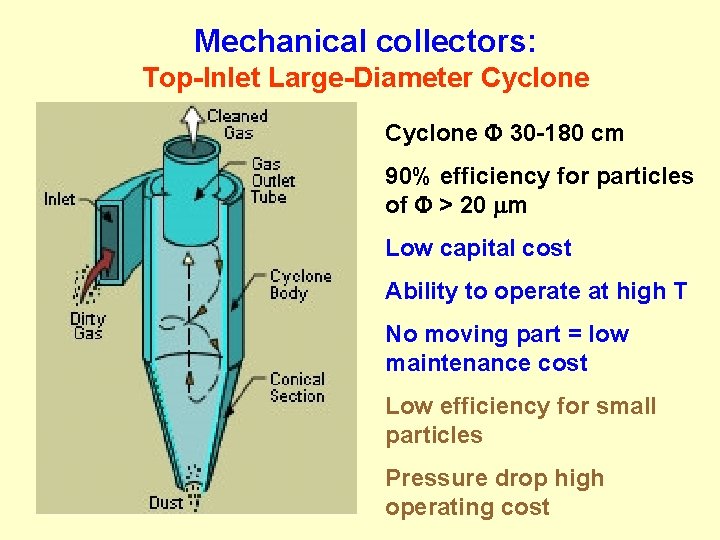
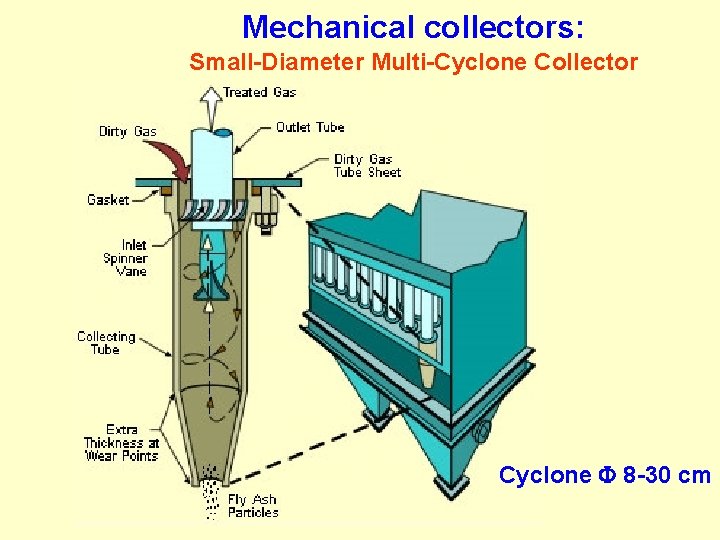
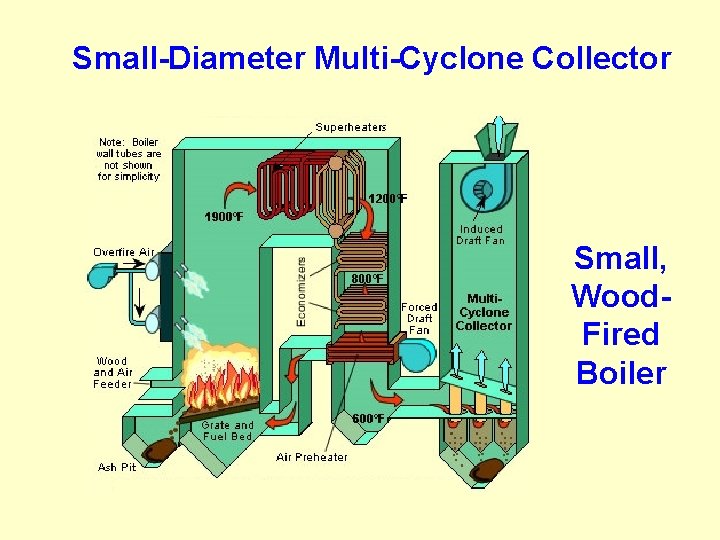
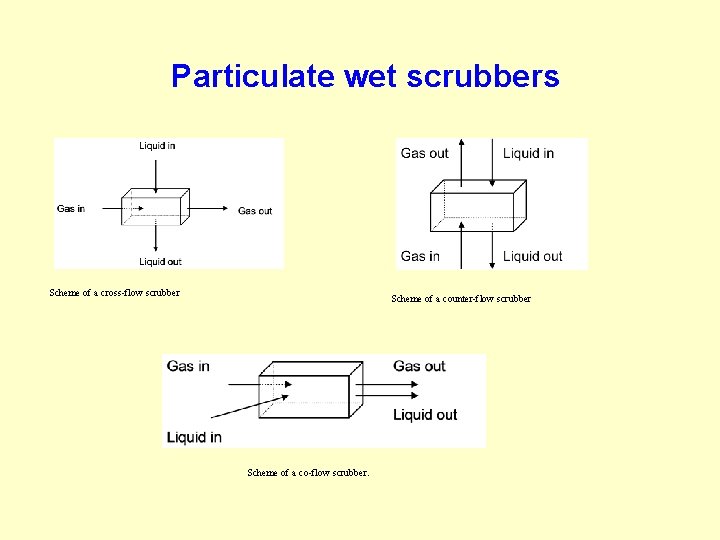
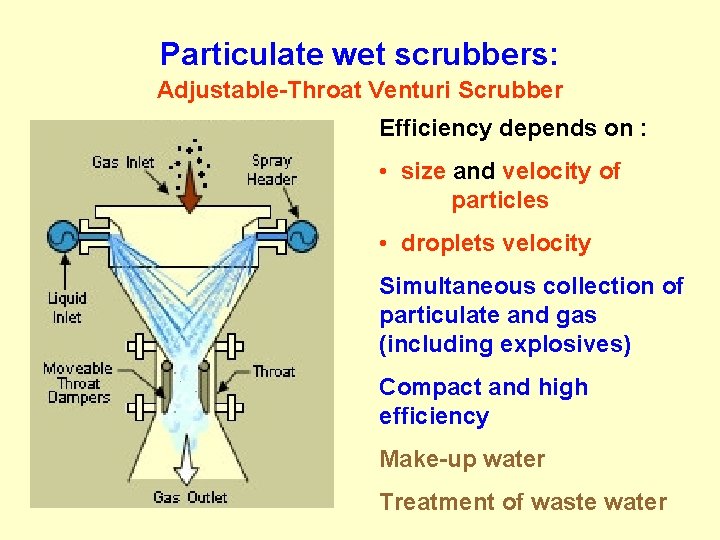
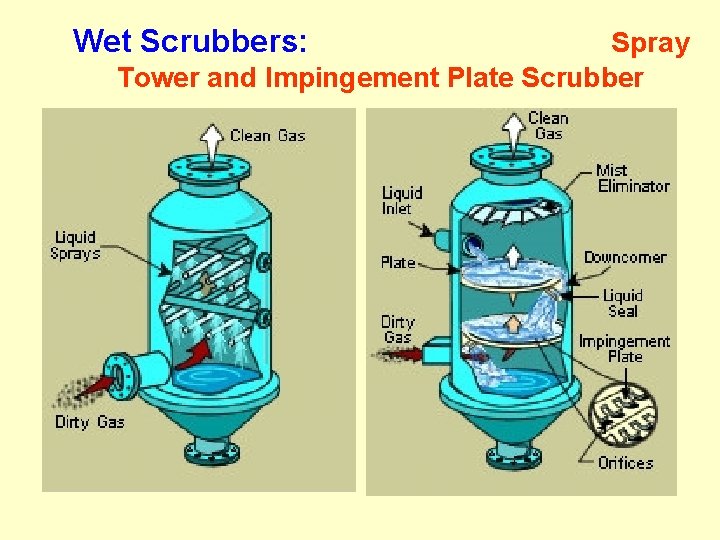
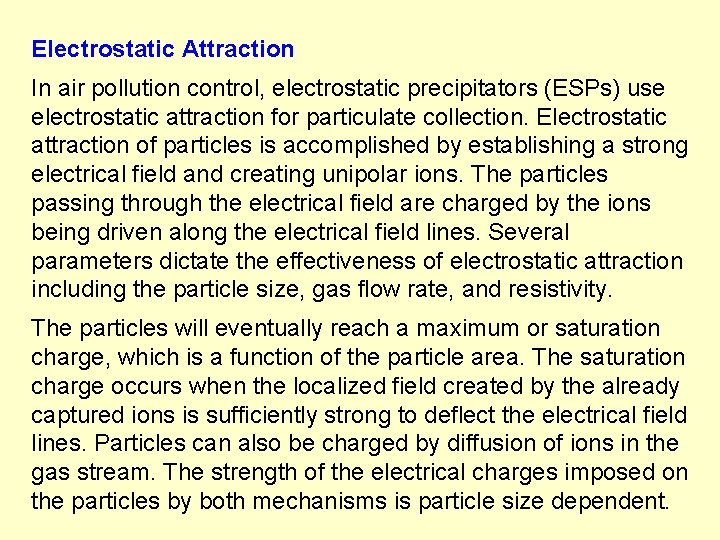
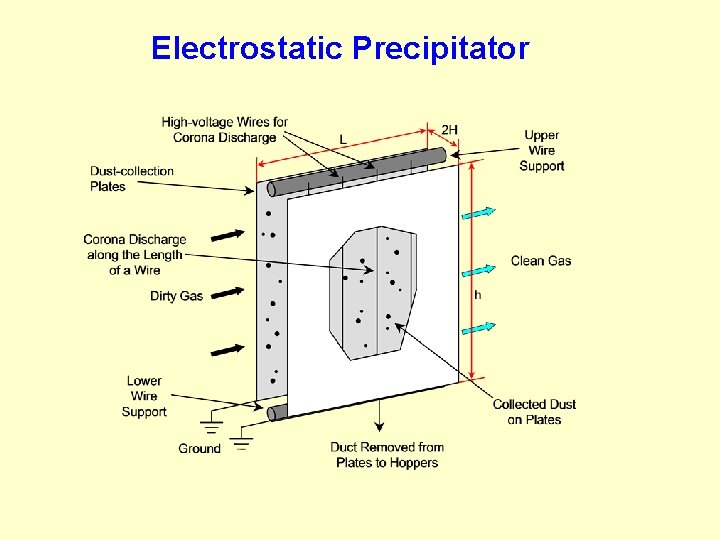
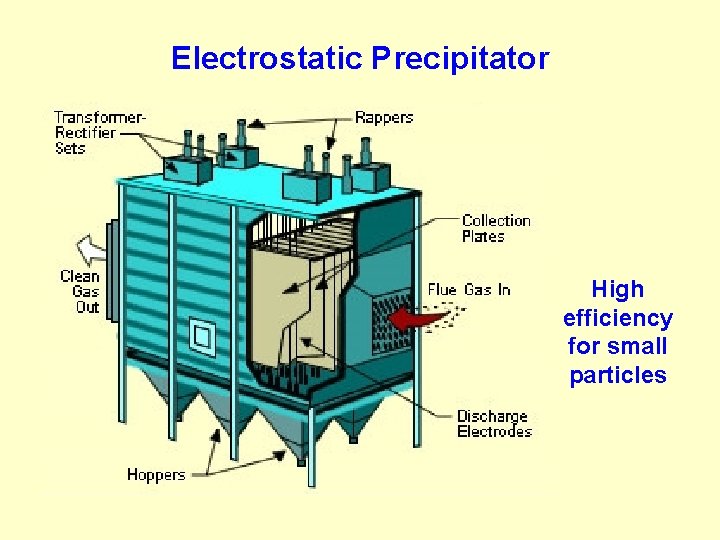
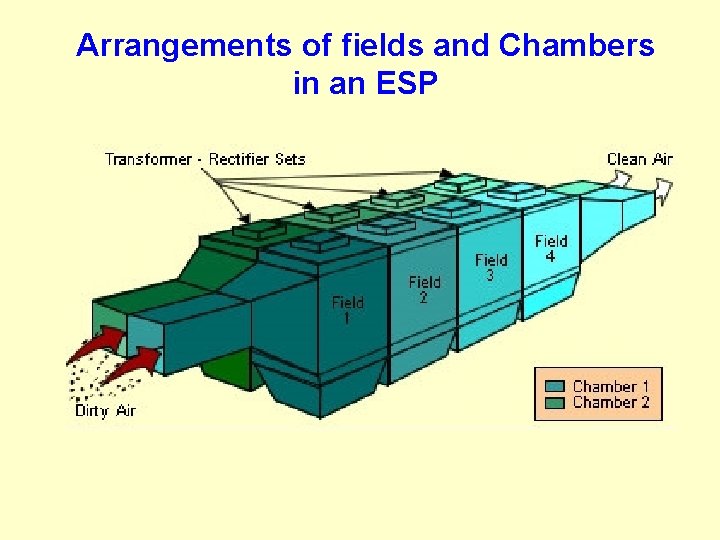
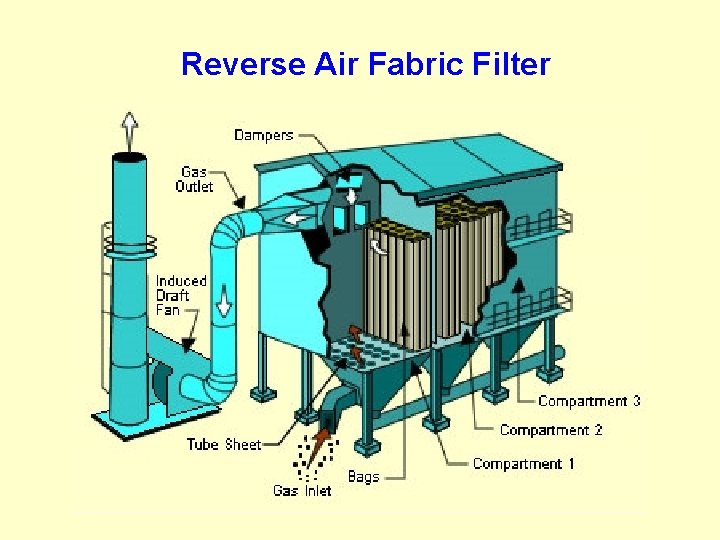
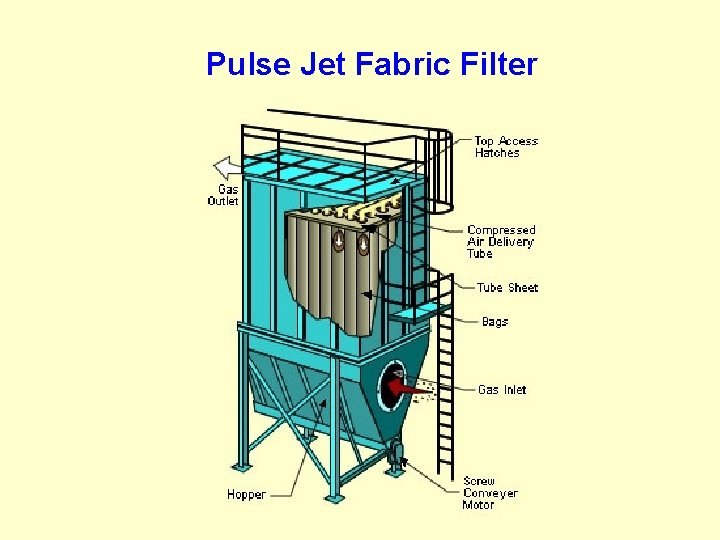
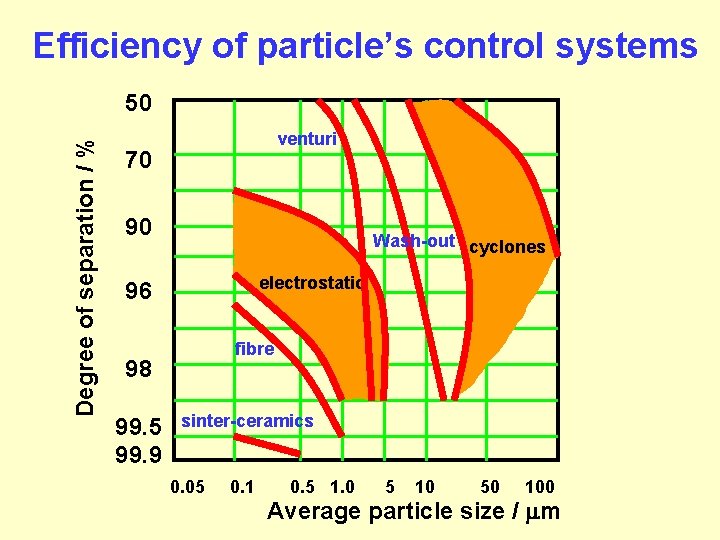
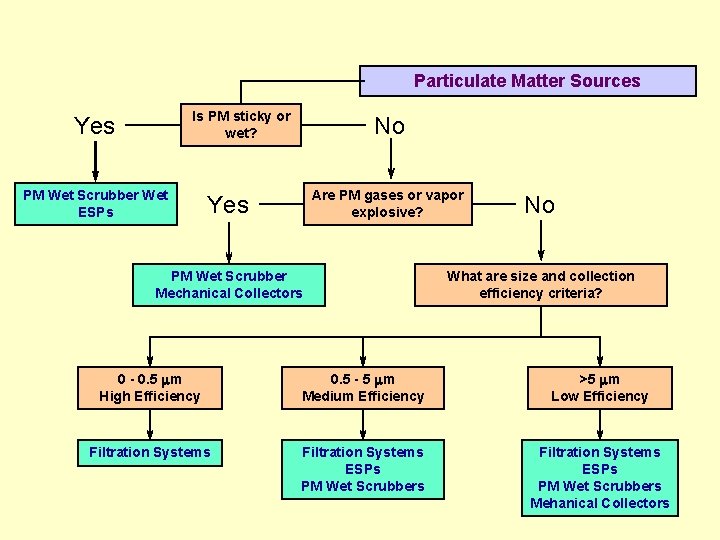
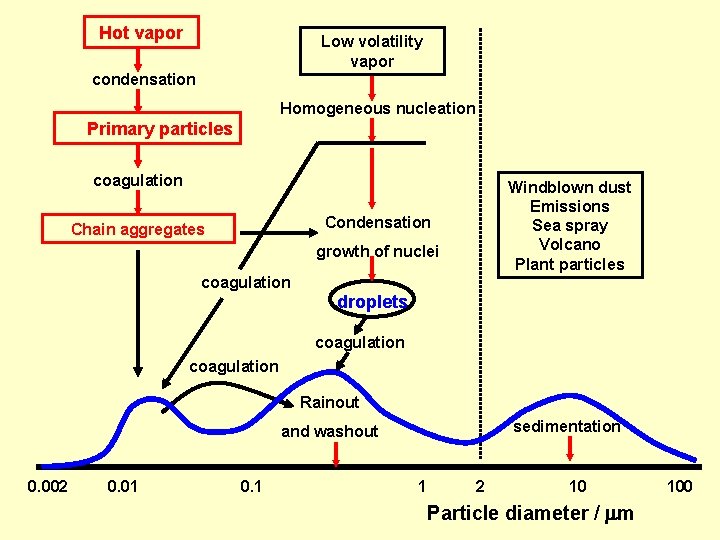
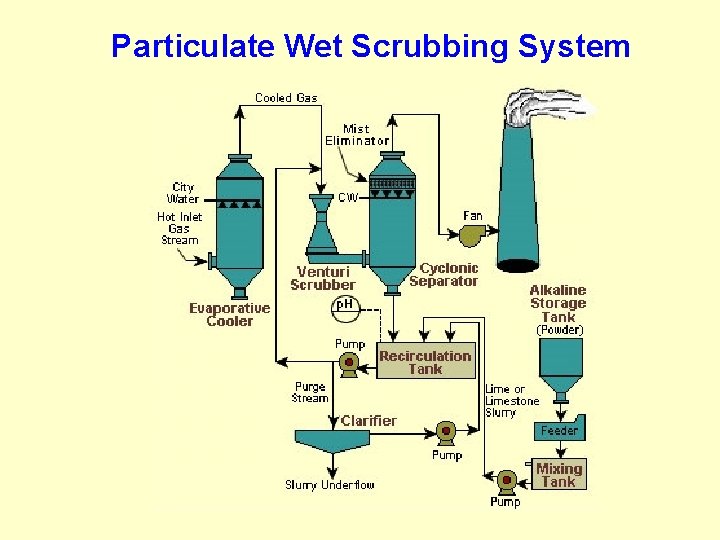
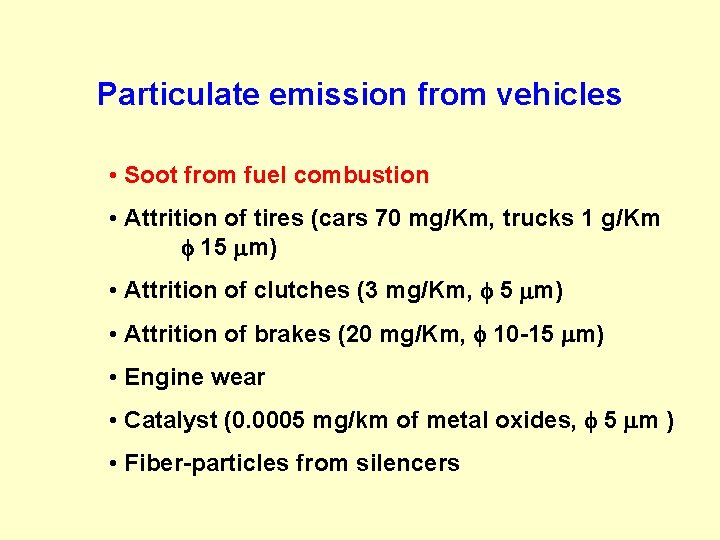
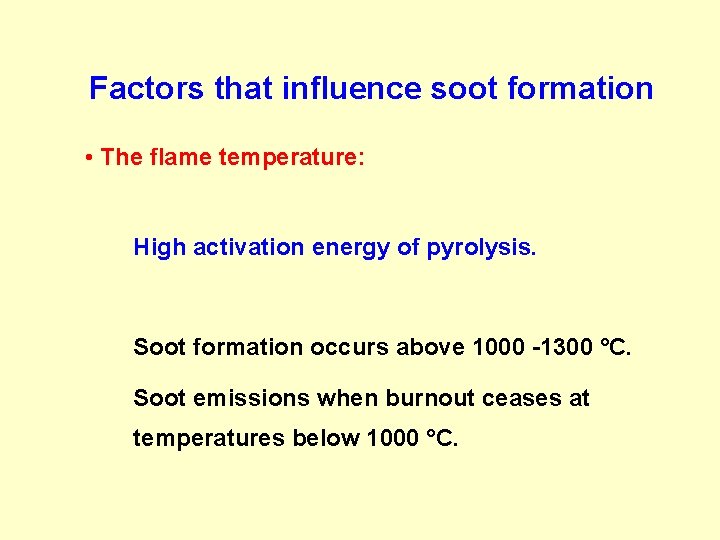
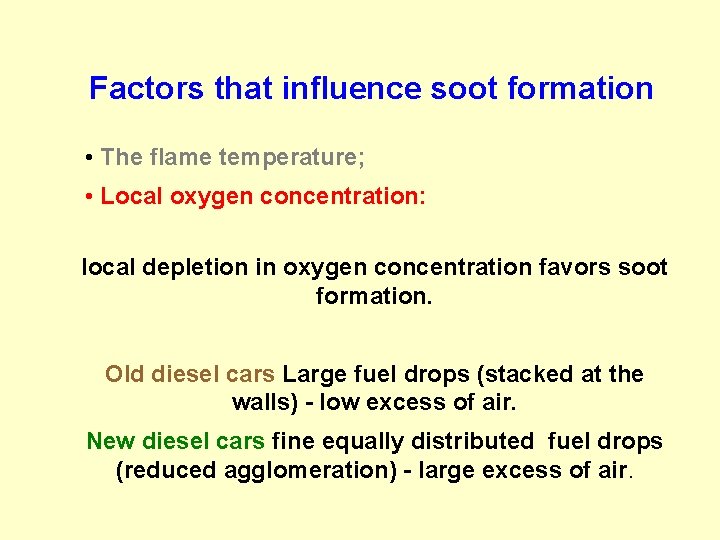
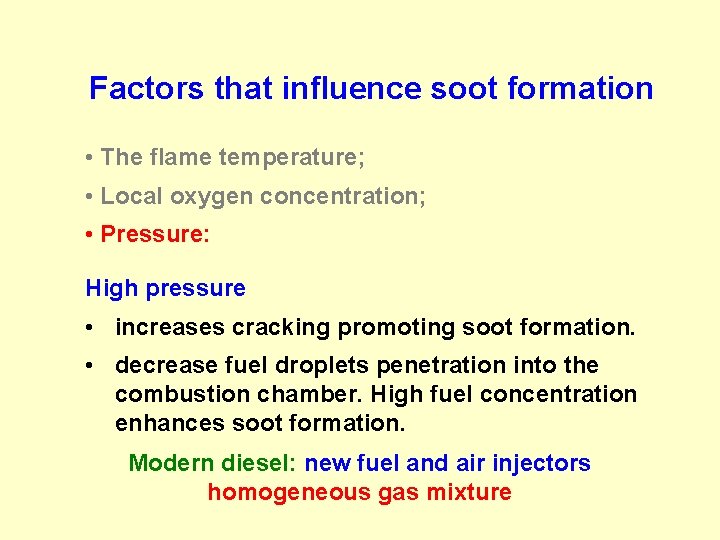
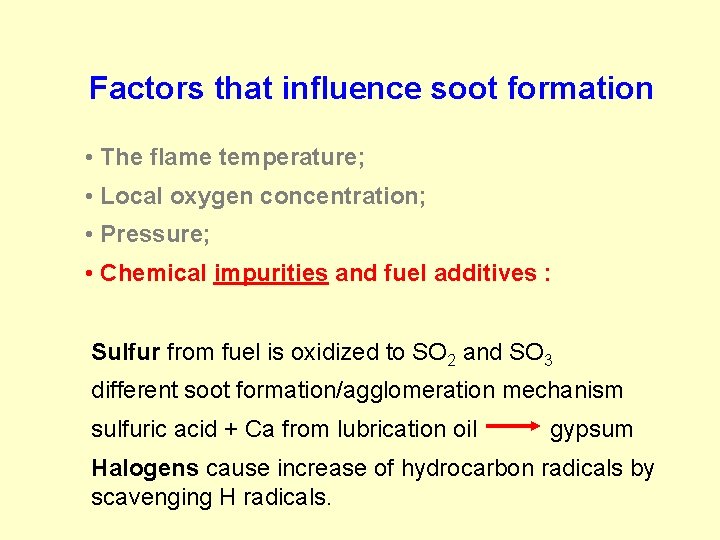
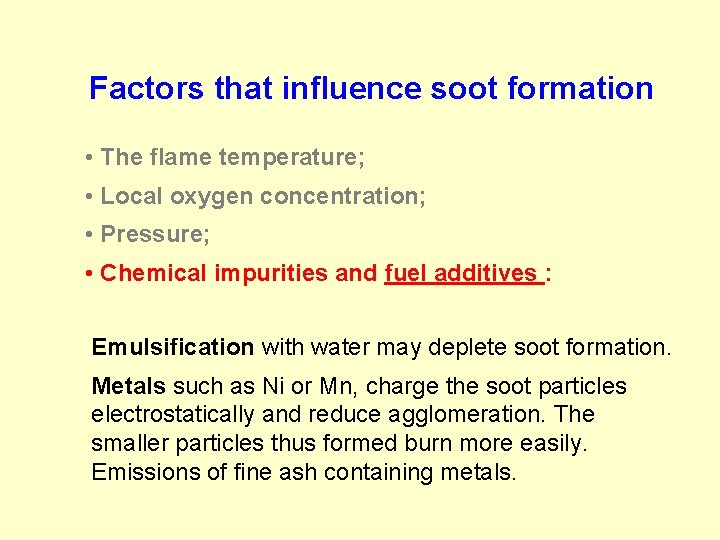
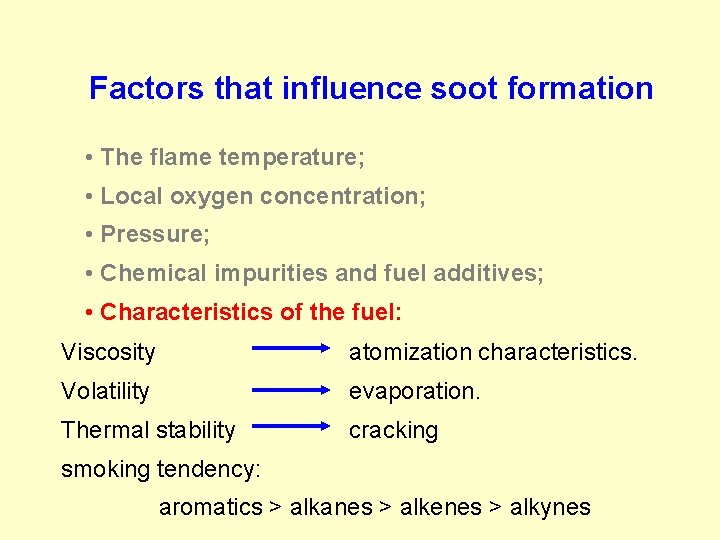
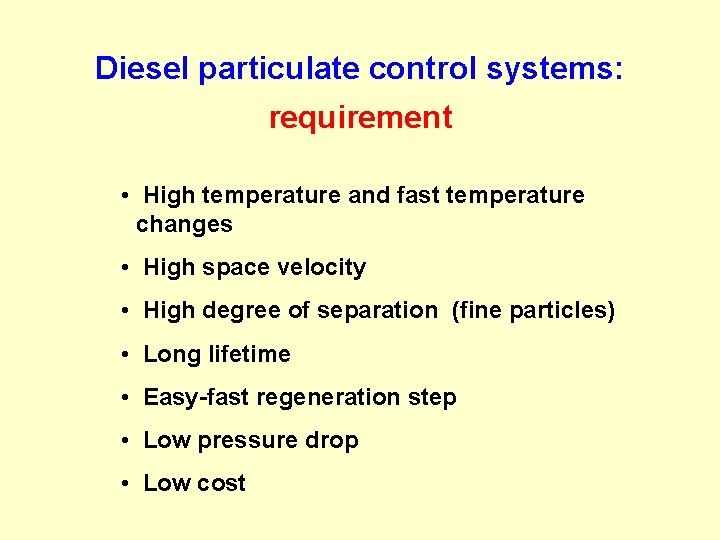
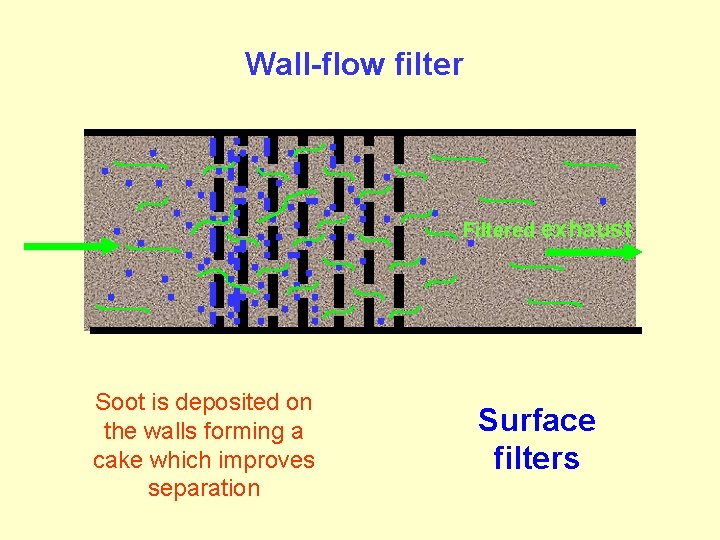
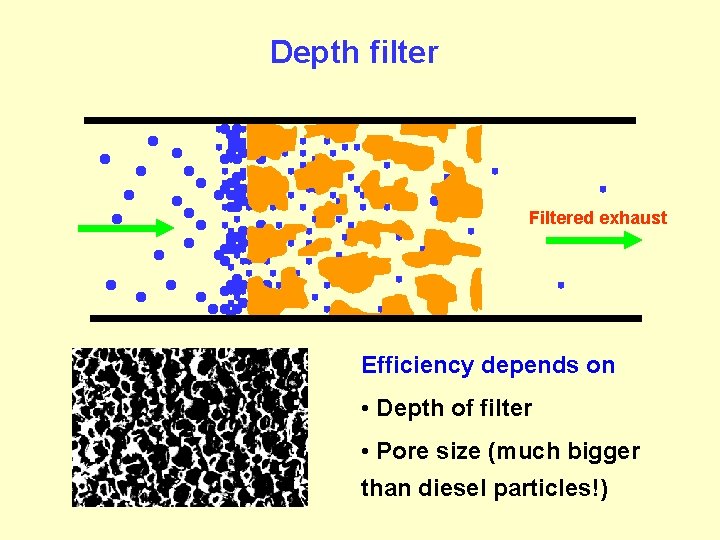
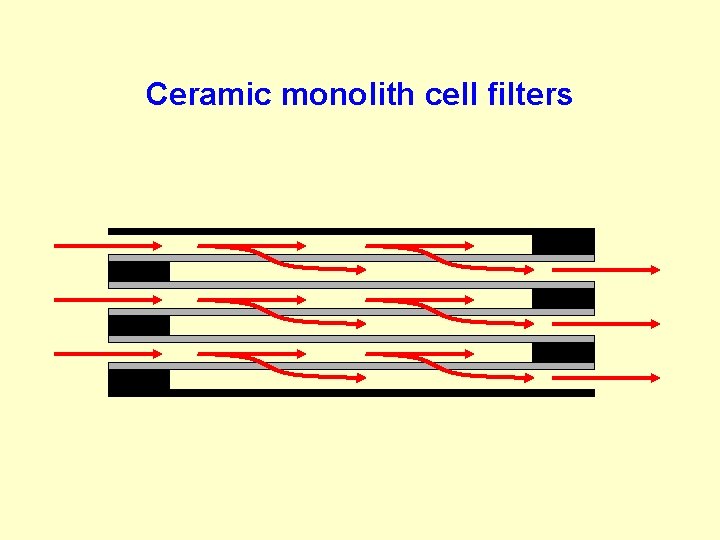
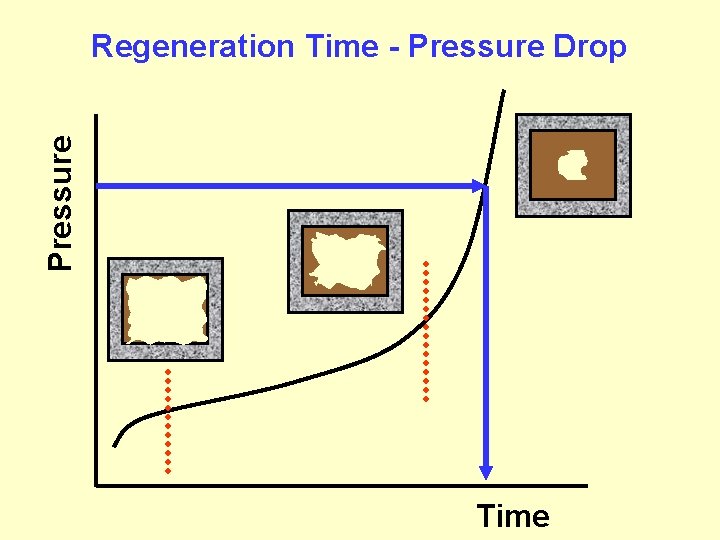
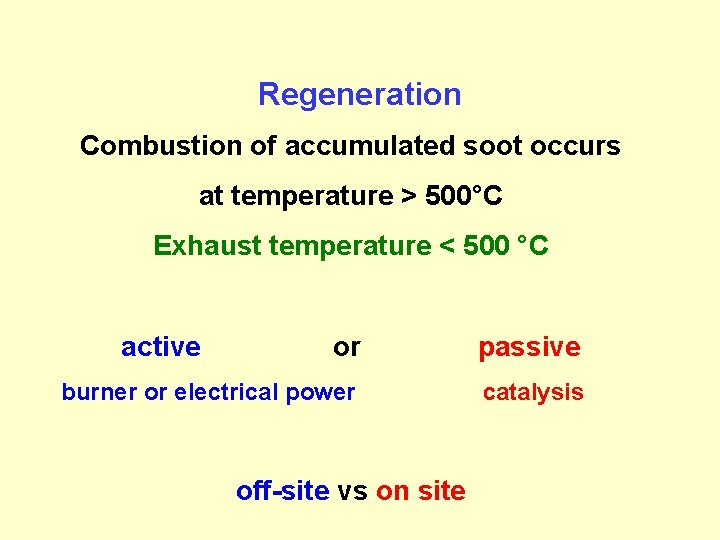
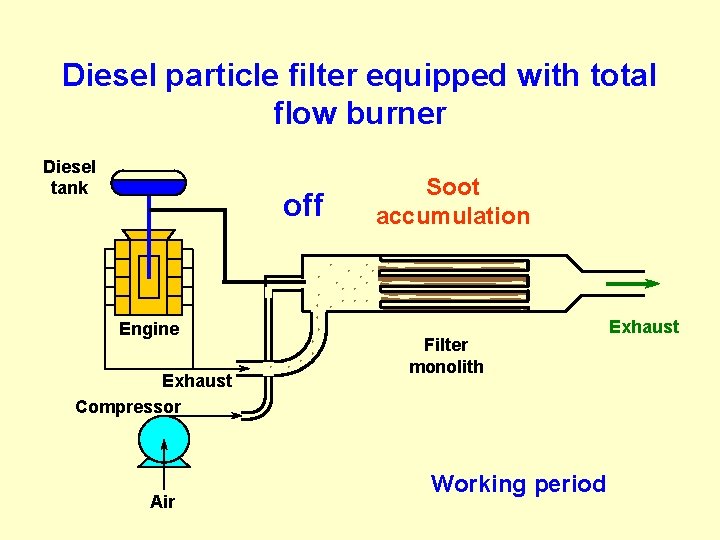
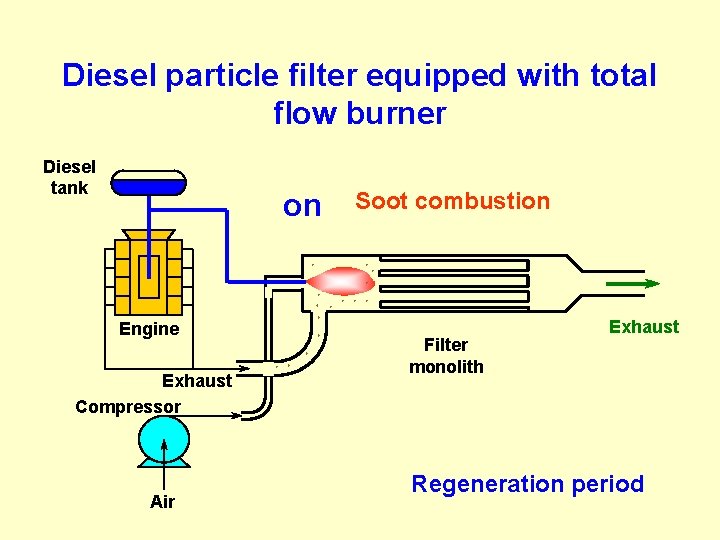
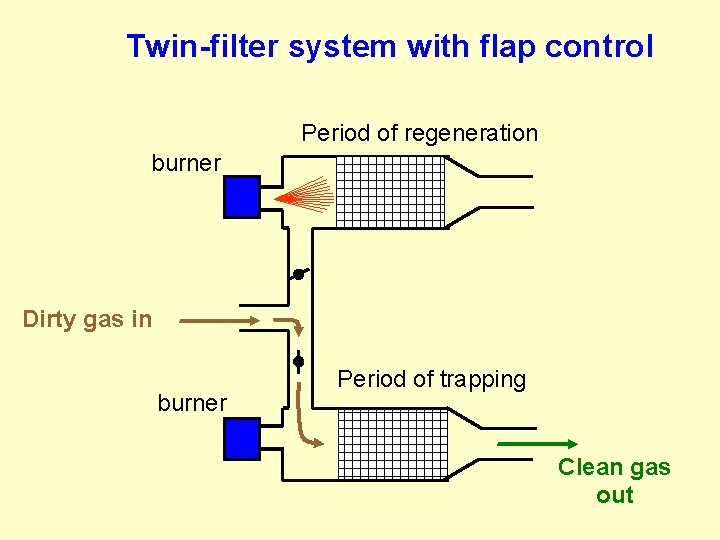
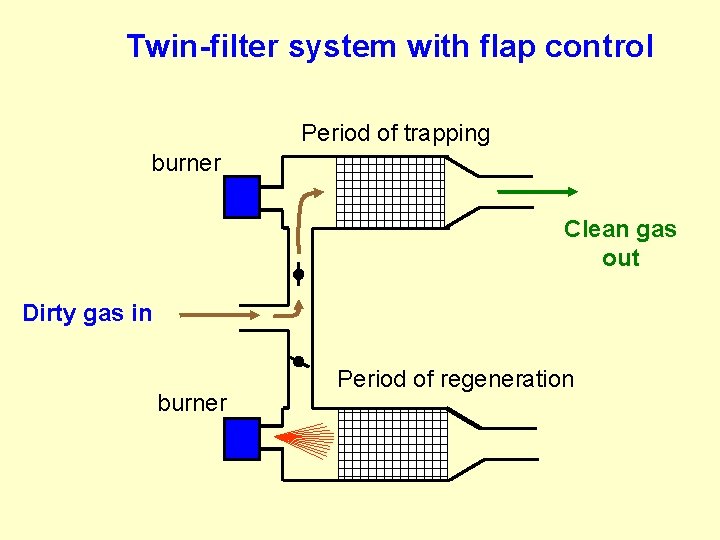
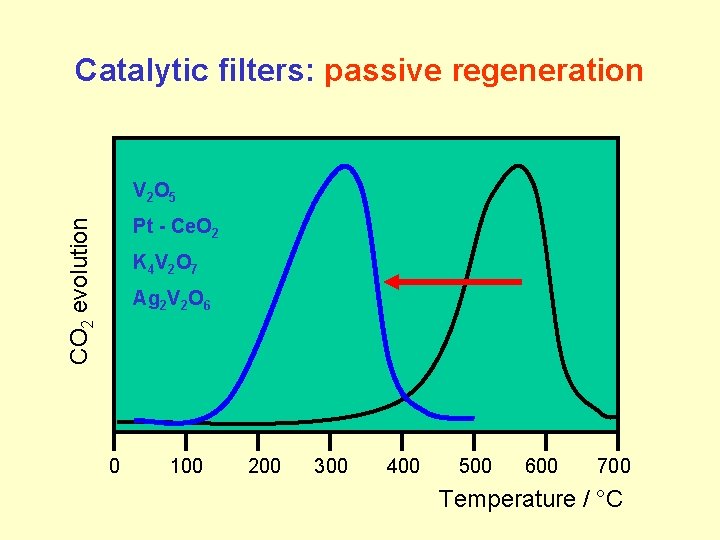
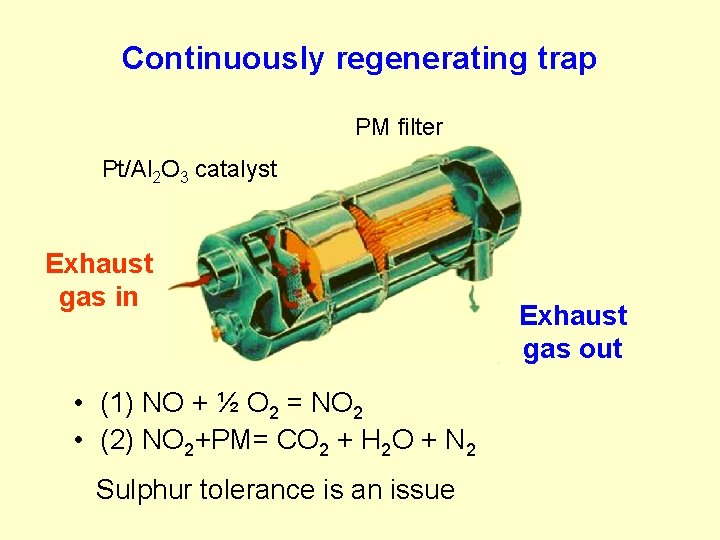
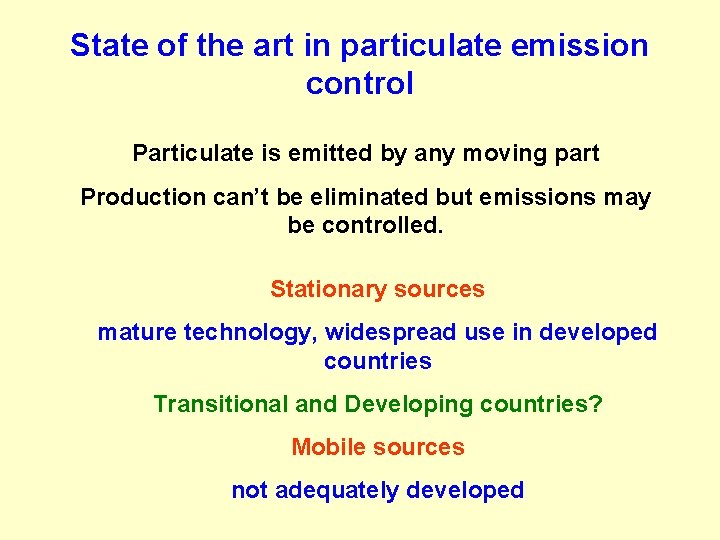
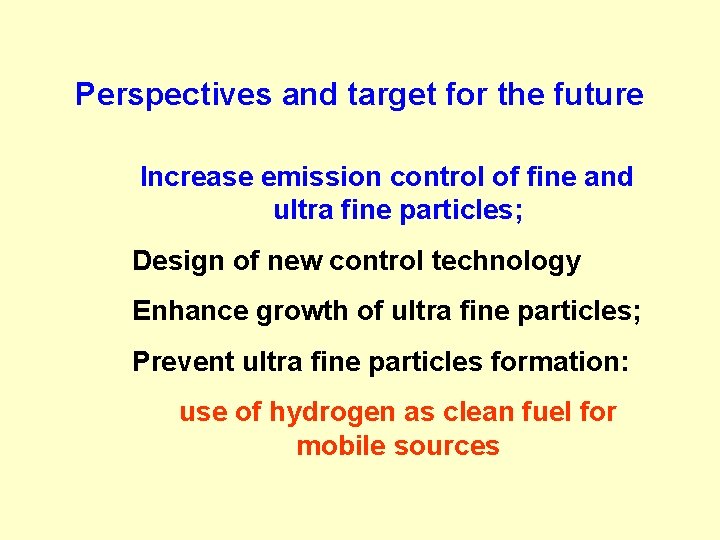
- Slides: 57
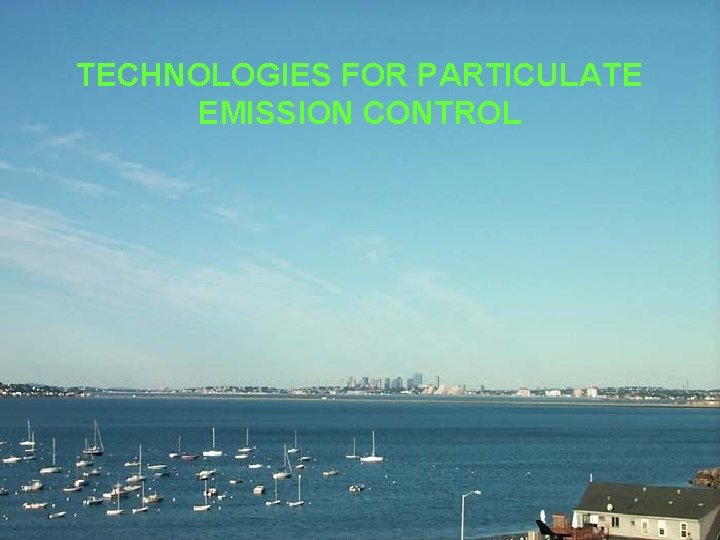
TECHNOLOGIES FOR PARTICULATE EMISSION CONTROL
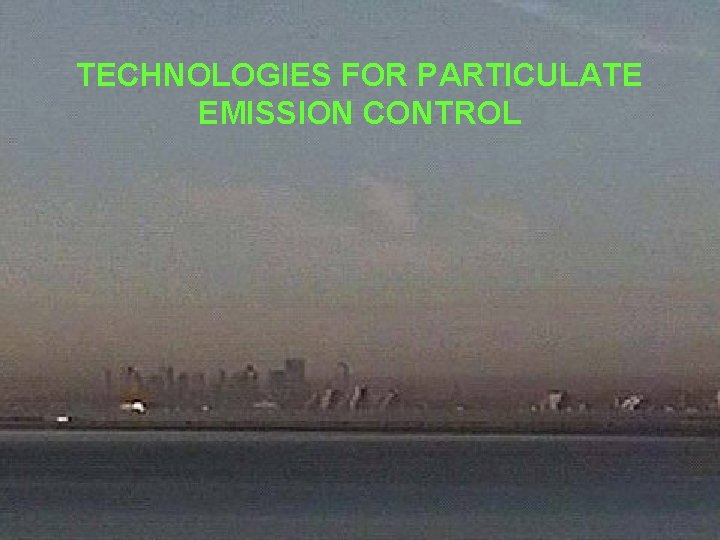
TECHNOLOGIES FOR PARTICULATE EMISSION CONTROL
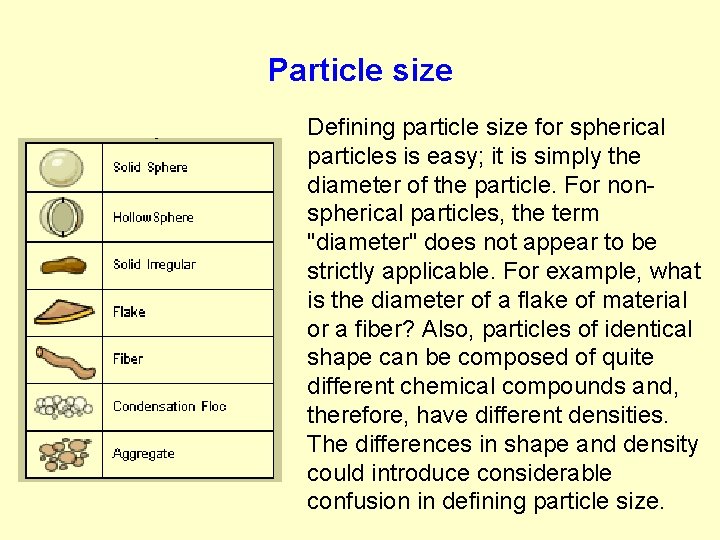
Particle size Defining particle size for spherical particles is easy; it is simply the diameter of the particle. For nonspherical particles, the term "diameter" does not appear to be strictly applicable. For example, what is the diameter of a flake of material or a fiber? Also, particles of identical shape can be composed of quite different chemical compounds and, therefore, have different densities. The differences in shape and density could introduce considerable confusion in defining particle size.
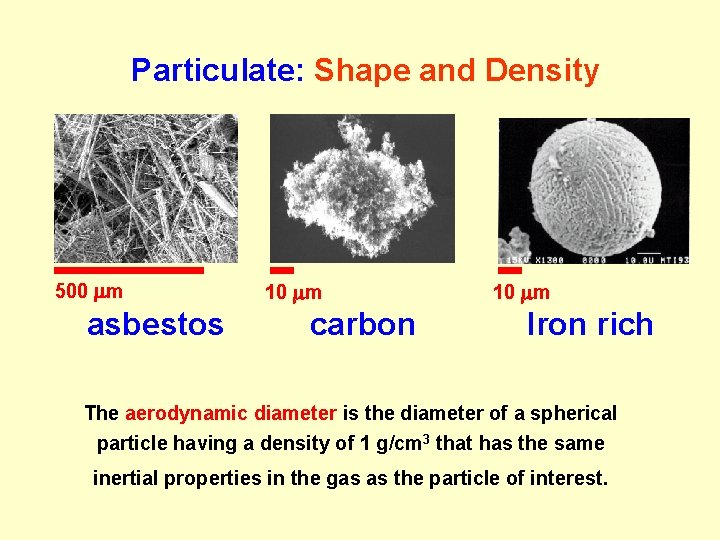
Particulate: Shape and Density 500 m asbestos 10 m carbon 10 m Iron rich The aerodynamic diameter is the diameter of a spherical particle having a density of 1 g/cm 3 that has the same inertial properties in the gas as the particle of interest.
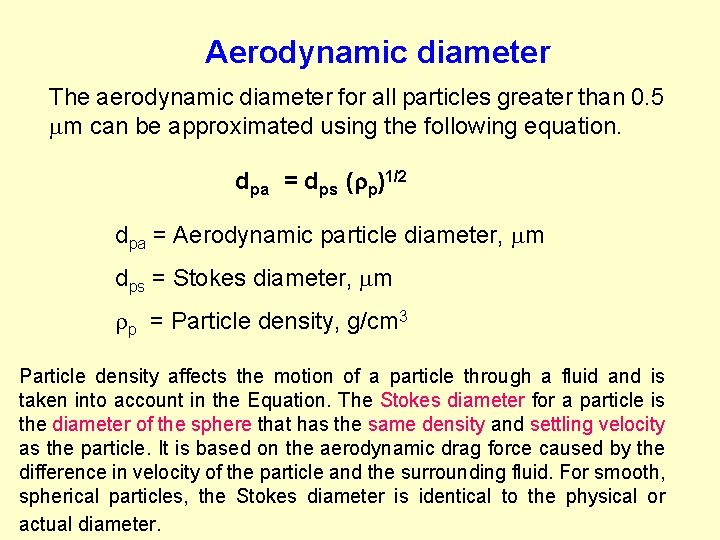
Aerodynamic diameter The aerodynamic diameter for all particles greater than 0. 5 mm can be approximated using the following equation. dpa = dps (rp)1/2 dpa = Aerodynamic particle diameter, mm dps = Stokes diameter, mm rp = Particle density, g/cm 3 Particle density affects the motion of a particle through a fluid and is taken into account in the Equation. The Stokes diameter for a particle is the diameter of the sphere that has the same density and settling velocity as the particle. It is based on the aerodynamic drag force caused by the difference in velocity of the particle and the surrounding fluid. For smooth, spherical particles, the Stokes diameter is identical to the physical or actual diameter.
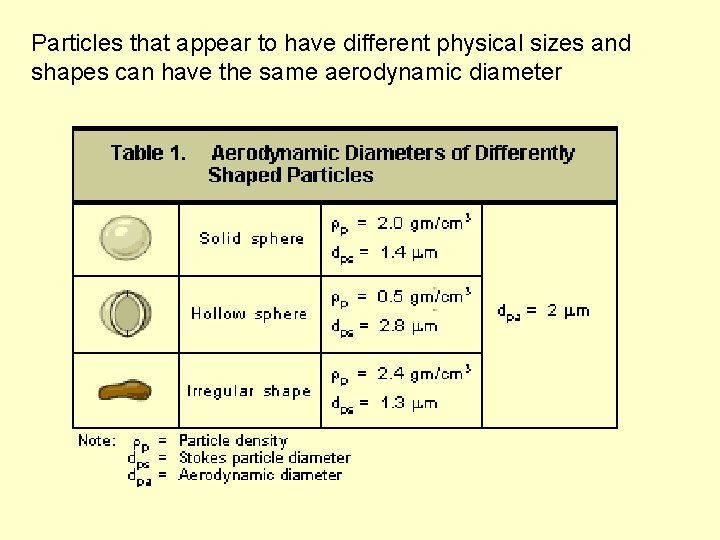
Particles that appear to have different physical sizes and shapes can have the same aerodynamic diameter
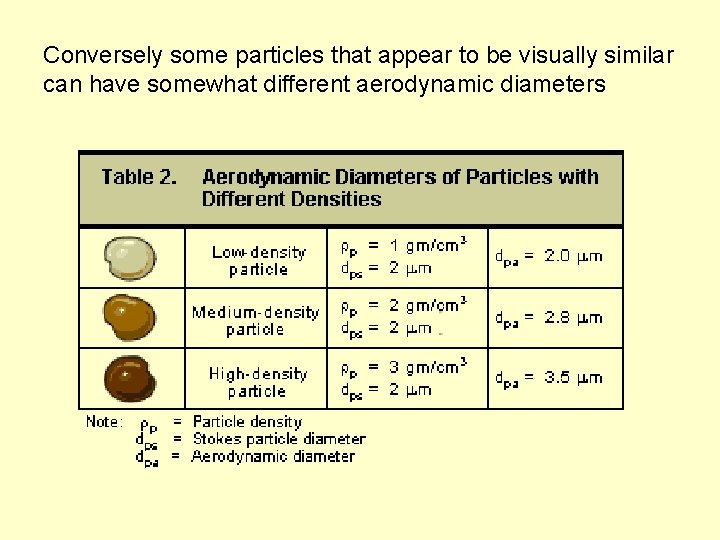
Conversely some particles that appear to be visually similar can have somewhat different aerodynamic diameters
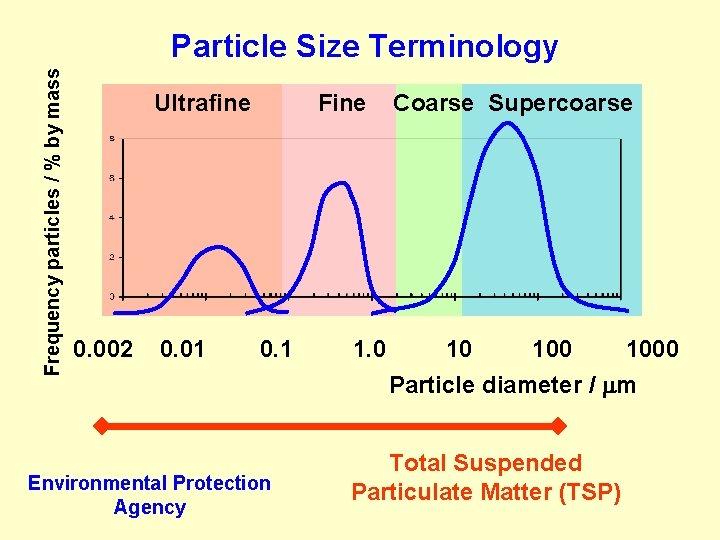
Frequency particles / % by mass Particle Size Terminology Ultrafine 0. 002 0. 01 Fine 0. 1 Environmental Protection Agency 1. 0 Coarse Supercoarse 10 1000 Particle diameter / m Total Suspended Particulate Matter (TSP)
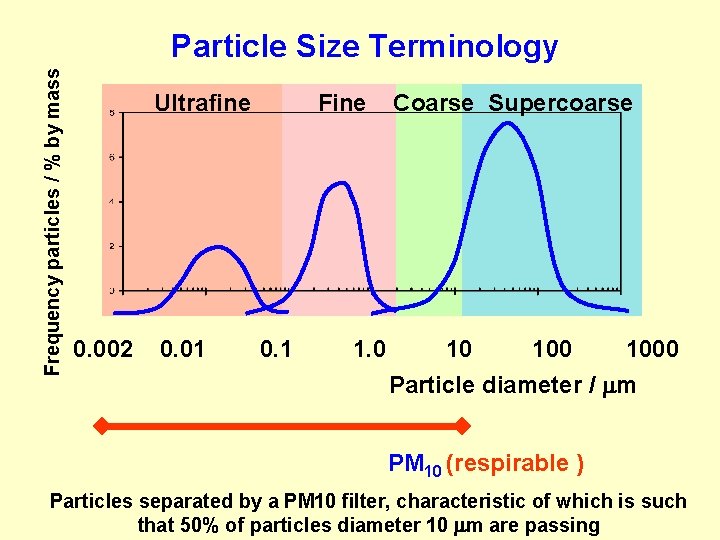
Frequency particles / % by mass Particle Size Terminology Ultrafine 0. 002 0. 01 Fine 0. 1 1. 0 Coarse Supercoarse 10 1000 Particle diameter / m PM 10 (respirable ) Particles separated by a PM 10 filter, characteristic of which is such that 50% of particles diameter 10 m are passing
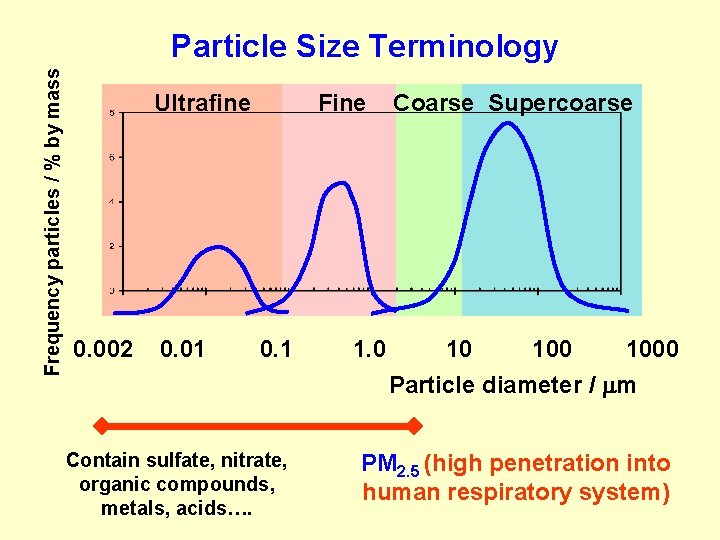
Frequency particles / % by mass Particle Size Terminology Ultrafine 0. 002 0. 01 Fine 0. 1 Contain sulfate, nitrate, organic compounds, metals, acids…. 1. 0 Coarse Supercoarse 10 1000 Particle diameter / m PM 2. 5 (high penetration into human respiratory system)
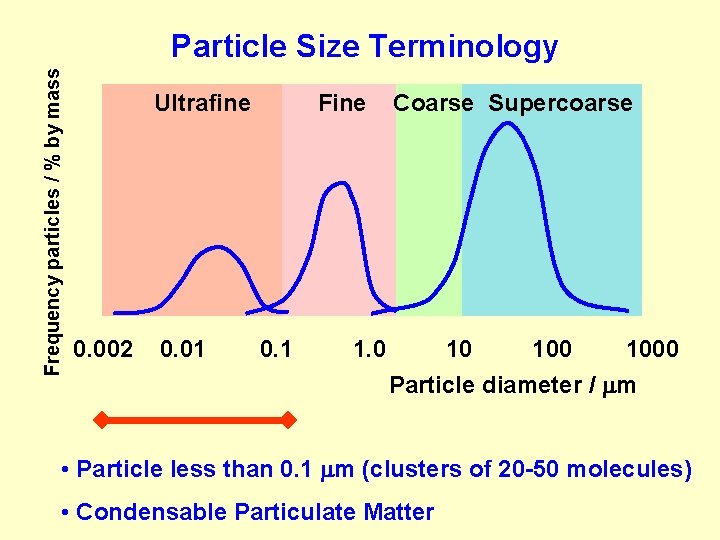
Frequency particles / % by mass Particle Size Terminology Ultrafine 0. 002 0. 01 Fine 0. 1 1. 0 Coarse Supercoarse 10 1000 Particle diameter / m • Particle less than 0. 1 m (clusters of 20 -50 molecules) • Condensable Particulate Matter
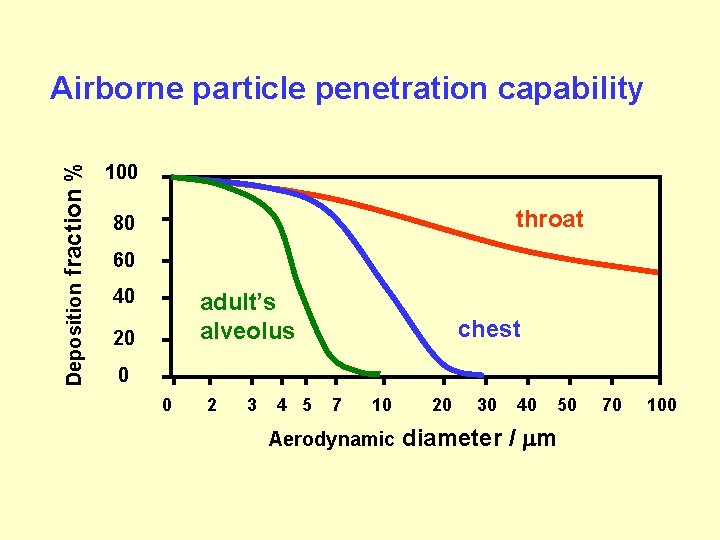
fraction % 100 Deposition Airborne particle penetration capability 40 throat 80 60 adult’s alveolus 20 chest 0 0 2 3 4 5 7 10 Aerodynamic 20 30 40 50 diameter / m 70 100
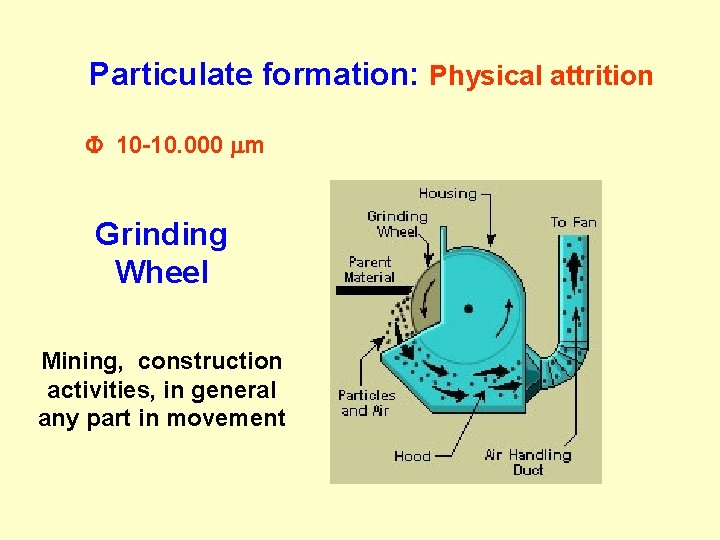
Particulate formation: Physical attrition F 10 -10. 000 m Grinding Wheel Mining, construction activities, in general any part in movement
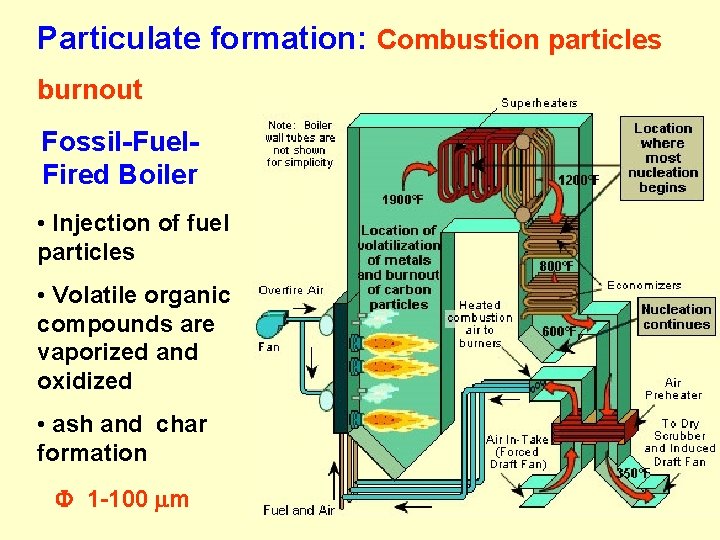
Particulate formation: Combustion particles burnout Fossil-Fuel. Fired Boiler • Injection of fuel particles • Volatile organic compounds are vaporized and oxidized • ash and char formation F 1 -100 m
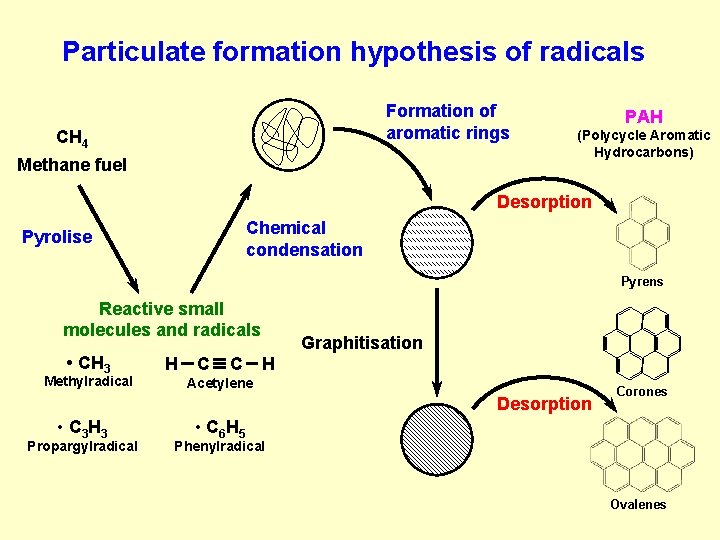
Particulate formation hypothesis of radicals Formation of aromatic rings CH 4 Methane fuel PAH (Polycycle Aromatic Hydrocarbons) Desorption Chemical condensation Pyrolise Pyrens Reactive small molecules and radicals • CH 3 Methylradical H C C Graphitisation H Acetylene Desorption • C 3 H 3 Propargylradical Corones • C 6 H 5 Phenylradical Ovalenes
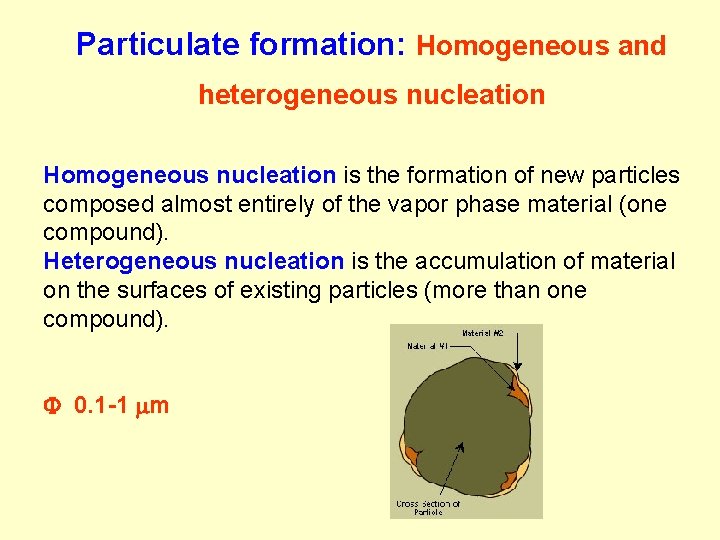
Particulate formation: Homogeneous and heterogeneous nucleation Homogeneous nucleation is the formation of new particles composed almost entirely of the vapor phase material (one compound). Heterogeneous nucleation is the accumulation of material on the surfaces of existing particles (more than one compound). F 0. 1 -1 m
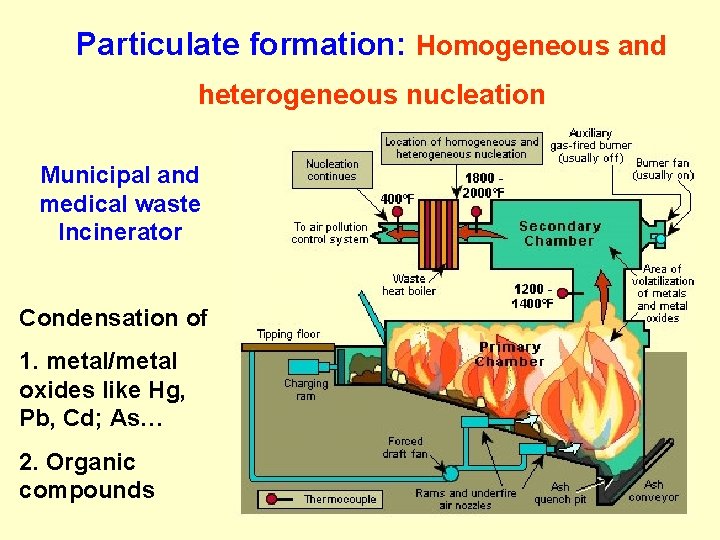
Particulate formation: Homogeneous and heterogeneous nucleation Municipal and medical waste Incinerator Condensation of 1. metal/metal oxides like Hg, Pb, Cd; As… 2. Organic compounds
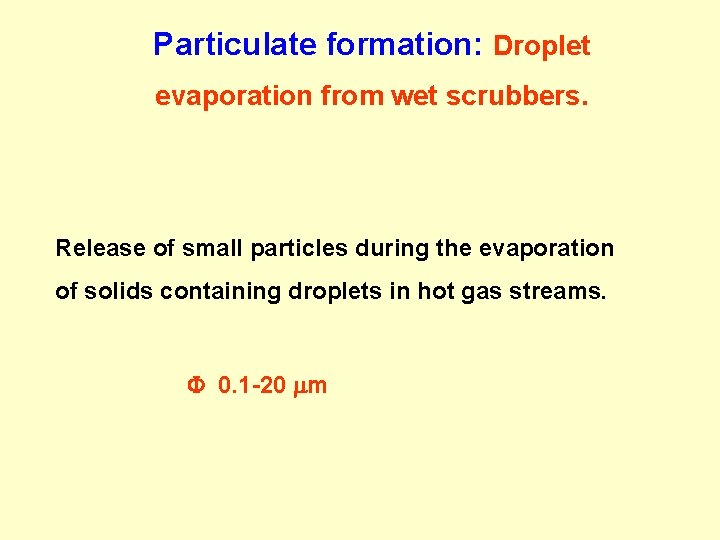
Particulate formation: Droplet evaporation from wet scrubbers. Release of small particles during the evaporation of solids containing droplets in hot gas streams. F 0. 1 -20 m
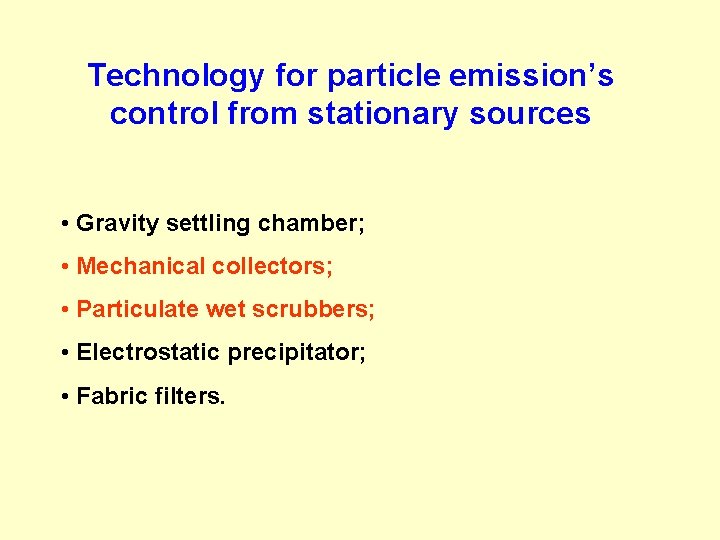
Technology for particle emission’s control from stationary sources • Gravity settling chamber; • Mechanical collectors; • Particulate wet scrubbers; • Electrostatic precipitator; • Fabric filters.
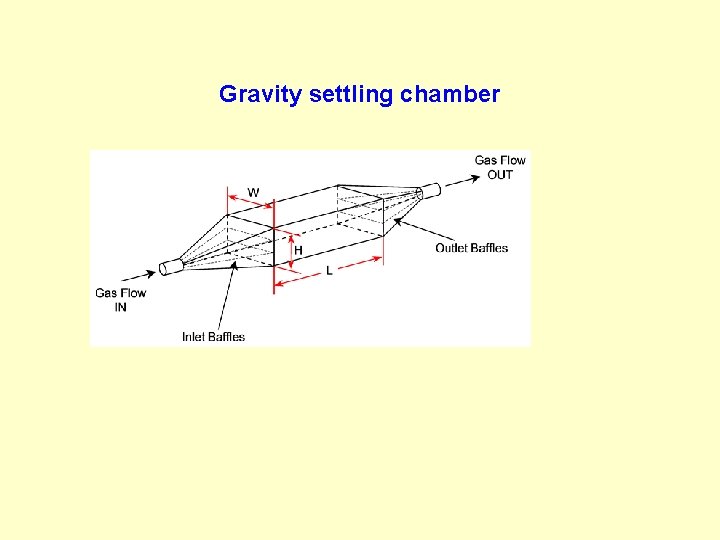
Gravity settling chamber
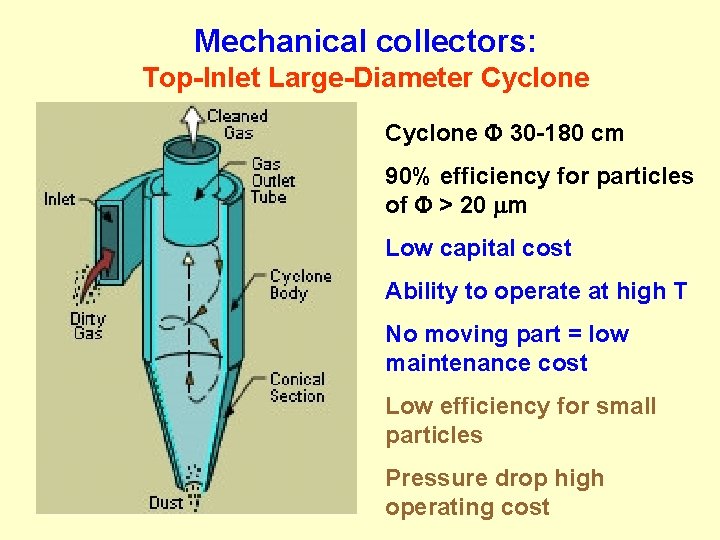
Mechanical collectors: Top-Inlet Large-Diameter Cyclone F 30 -180 cm 90% efficiency for particles of F > 20 m Low capital cost Ability to operate at high T No moving part = low maintenance cost Low efficiency for small particles Pressure drop high operating cost
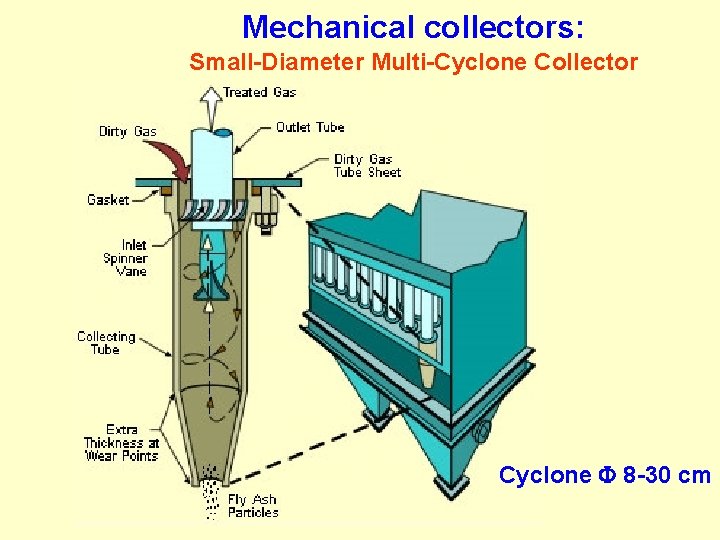
Mechanical collectors: Small-Diameter Multi-Cyclone Collector Cyclone F 8 -30 cm
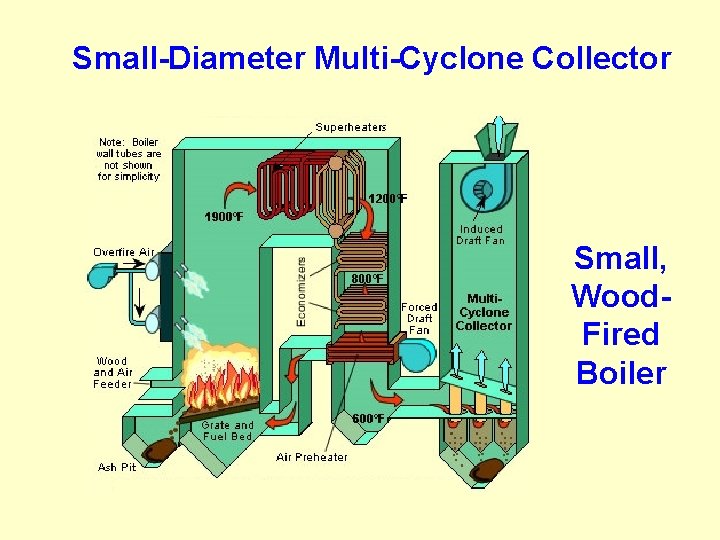
Small-Diameter Multi-Cyclone Collector Small, Wood. Fired Boiler
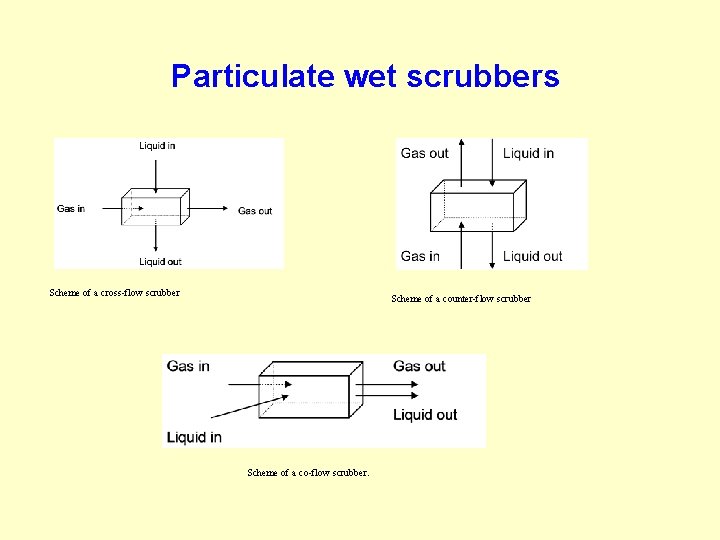
Particulate wet scrubbers Scheme of a cross-flow scrubber Scheme of a counter-flow scrubber Scheme of a co-flow scrubber.
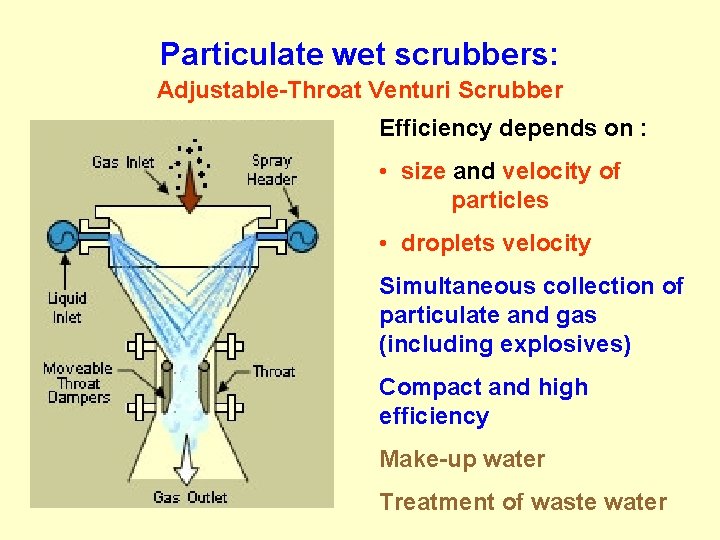
Particulate wet scrubbers: Adjustable-Throat Venturi Scrubber Efficiency depends on : • size and velocity of particles • droplets velocity Simultaneous collection of particulate and gas (including explosives) Compact and high efficiency Make-up water Treatment of waste water
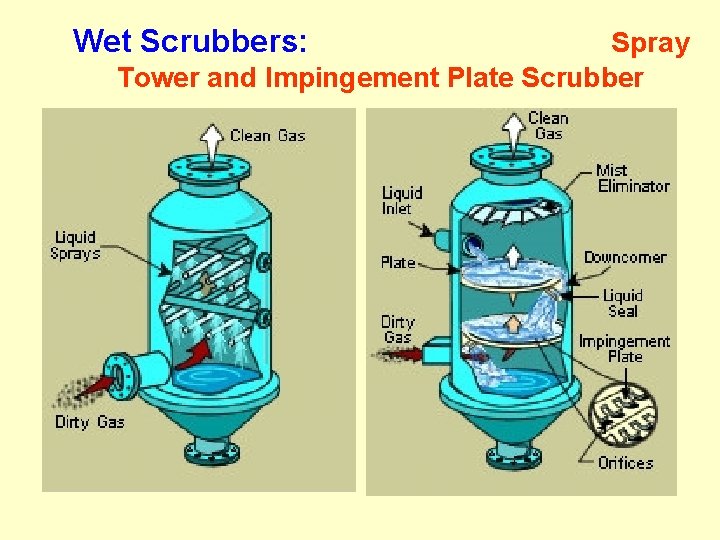
Wet Scrubbers: Spray Tower and Impingement Plate Scrubber
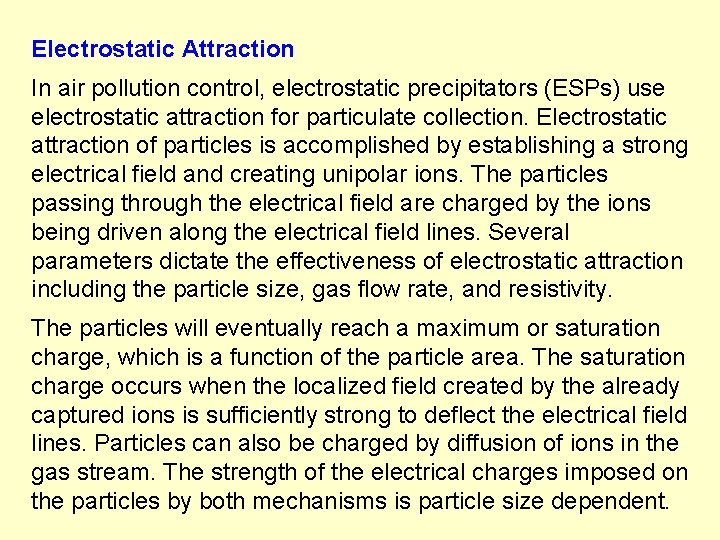
Electrostatic Attraction In air pollution control, electrostatic precipitators (ESPs) use electrostatic attraction for particulate collection. Electrostatic attraction of particles is accomplished by establishing a strong electrical field and creating unipolar ions. The particles passing through the electrical field are charged by the ions being driven along the electrical field lines. Several parameters dictate the effectiveness of electrostatic attraction including the particle size, gas flow rate, and resistivity. The particles will eventually reach a maximum or saturation charge, which is a function of the particle area. The saturation charge occurs when the localized field created by the already captured ions is sufficiently strong to deflect the electrical field lines. Particles can also be charged by diffusion of ions in the gas stream. The strength of the electrical charges imposed on the particles by both mechanisms is particle size dependent.
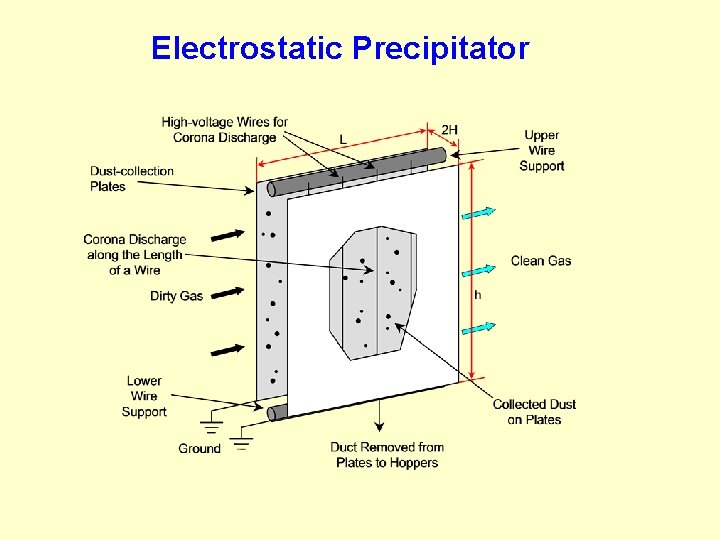
Electrostatic Precipitator
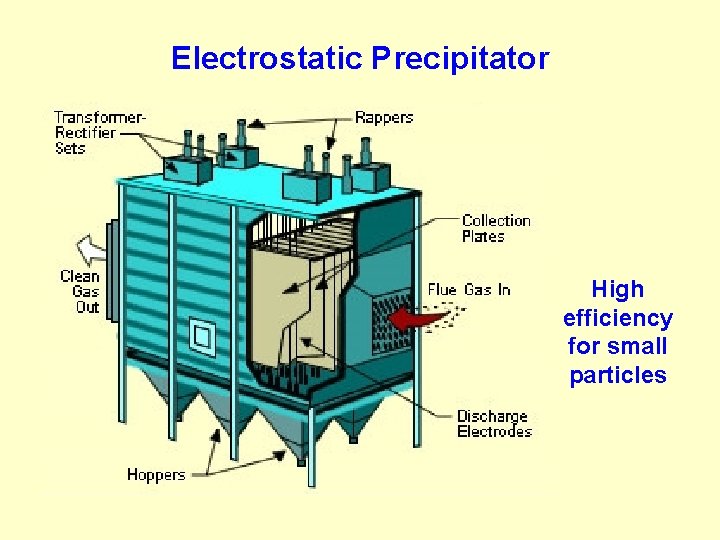
Electrostatic Precipitator High efficiency for small particles
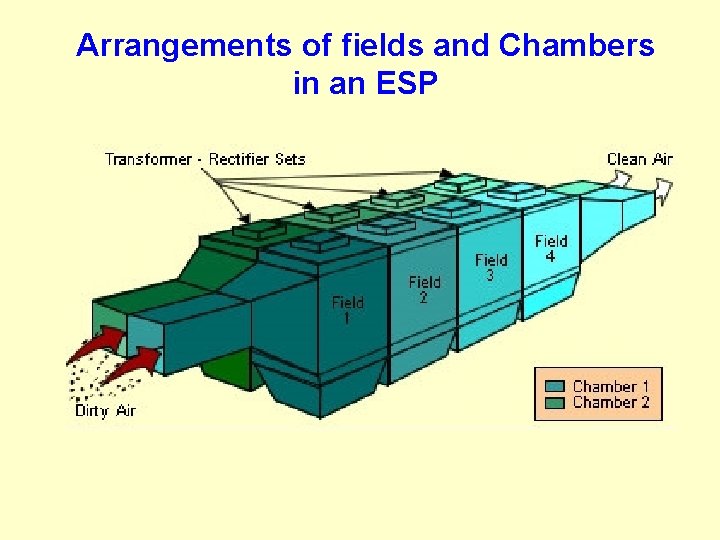
Arrangements of fields and Chambers in an ESP
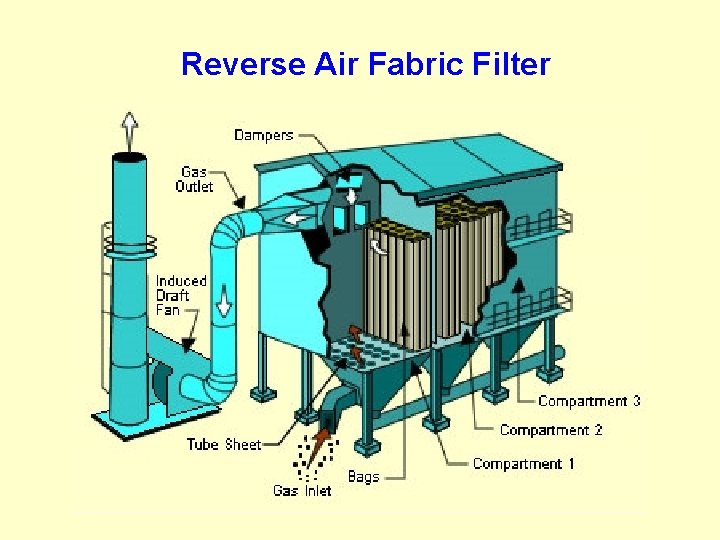
Reverse Air Fabric Filter
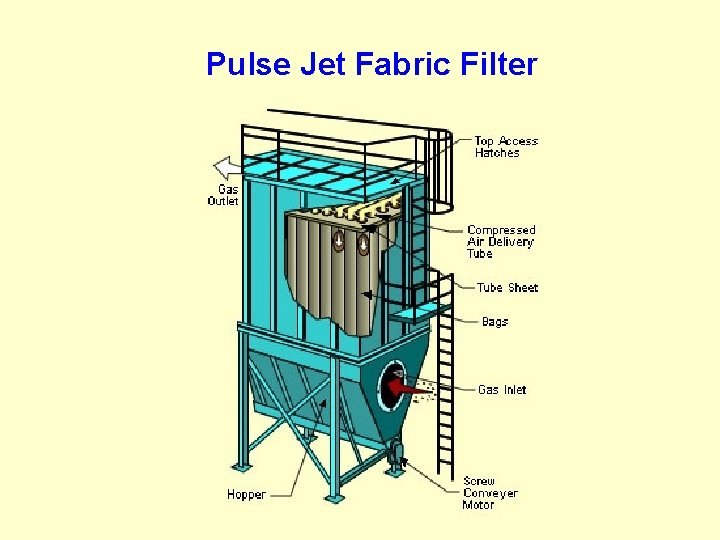
Pulse Jet Fabric Filter
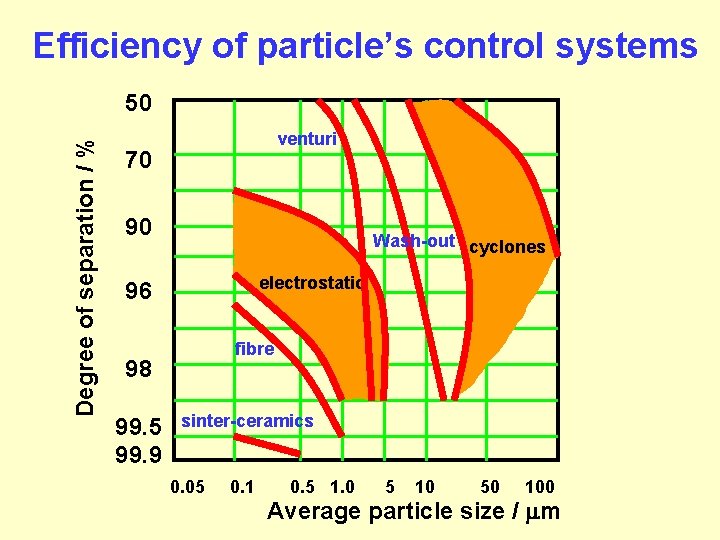
Efficiency of particle’s control systems Degree of separation / % 50 venturi 70 cyclotron 90 Wash-out cyclones electrostatic 96 fibre 98 99. 5 99. 9 sinter-ceramics 0. 05 0. 1 0. 5 1. 0 5 10 50 100 Average particle size / m
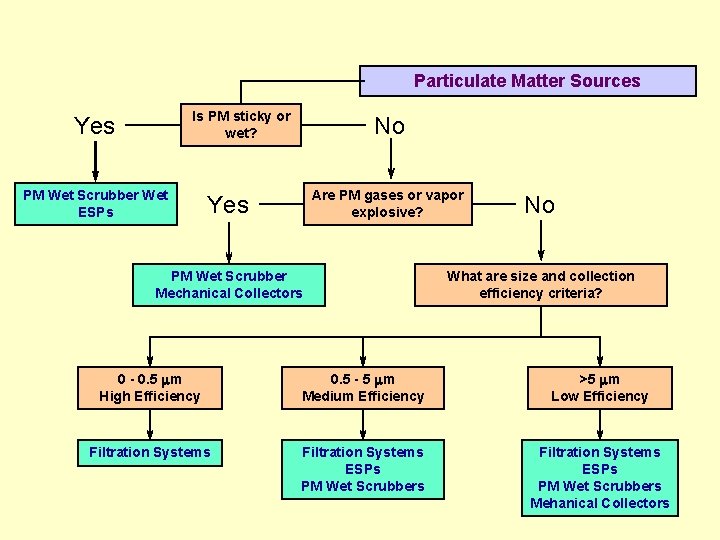
Particulate Matter Sources Is PM sticky or wet? Yes PM Wet Scrubber Wet ESPs No Are PM gases or vapor explosive? Yes PM Wet Scrubber Mechanical Collectors No What are size and collection efficiency criteria? 0 - 0. 5 m High Efficiency 0. 5 - 5 m Medium Efficiency >5 m Low Efficiency Filtration Systems ESPs PM Wet Scrubbers Mehanical Collectors
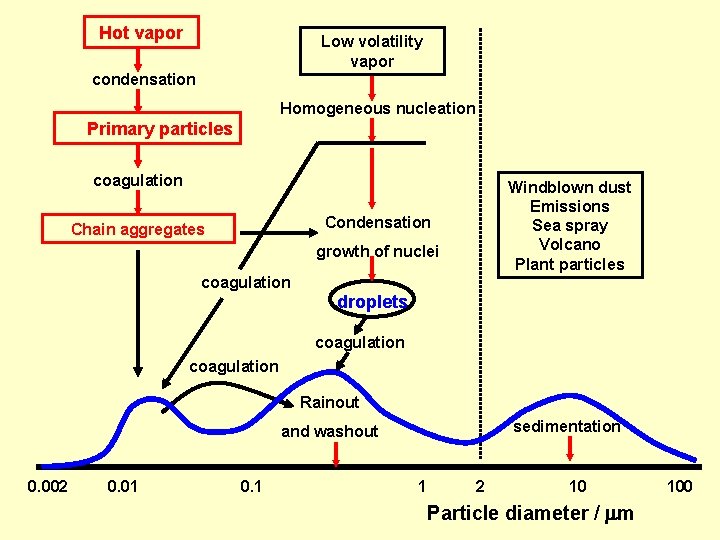
Hot vapor Low volatility vapor condensation Homogeneous nucleation Primary particles coagulation Windblown dust Emissions Sea spray Volcano Plant particles Condensation Chain aggregates growth of nuclei coagulation droplets coagulation Rainout sedimentation and washout 0. 002 0. 01 0. 1 1 2 10 Particle diameter / m 100
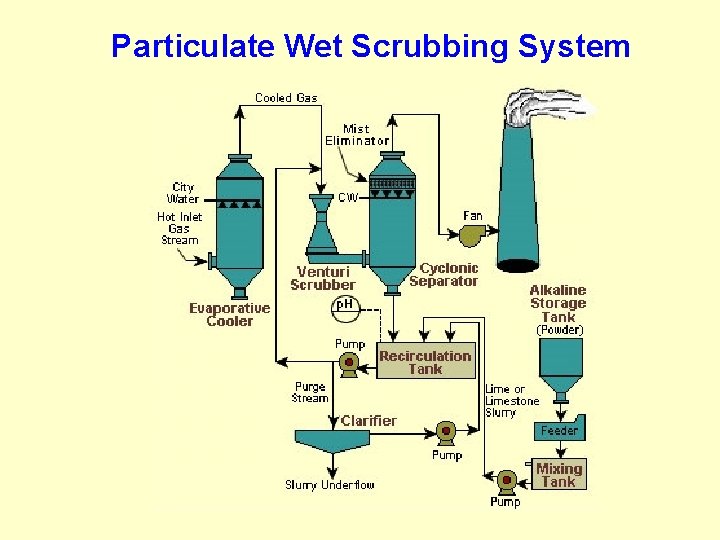
Particulate Wet Scrubbing System
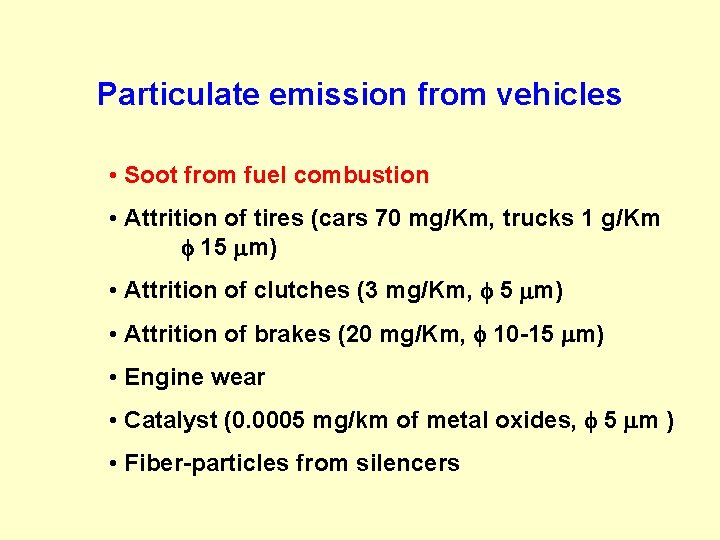
Particulate emission from vehicles • Soot from fuel combustion • Attrition of tires (cars 70 mg/Km, trucks 1 g/Km f 15 m) • Attrition of clutches (3 mg/Km, f 5 m) • Attrition of brakes (20 mg/Km, f 10 -15 m) • Engine wear • Catalyst (0. 0005 mg/km of metal oxides, f 5 m ) • Fiber-particles from silencers
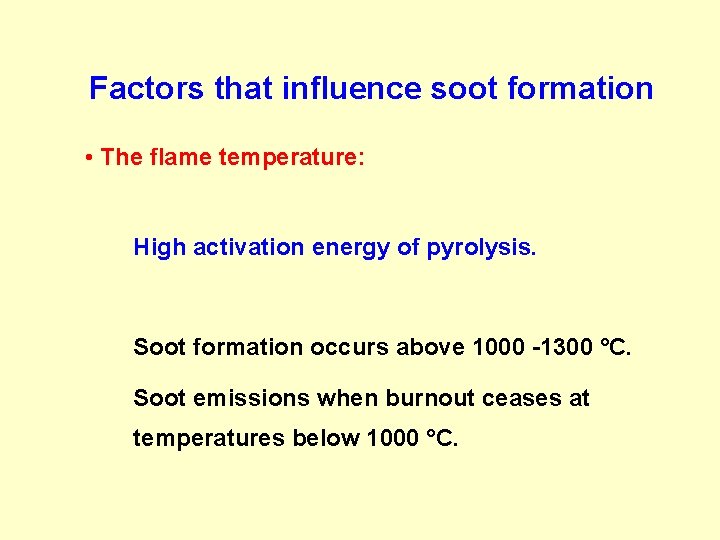
Factors that influence soot formation • The flame temperature: High activation energy of pyrolysis. Soot formation occurs above 1000 -1300 °C. Soot emissions when burnout ceases at temperatures below 1000 °C.
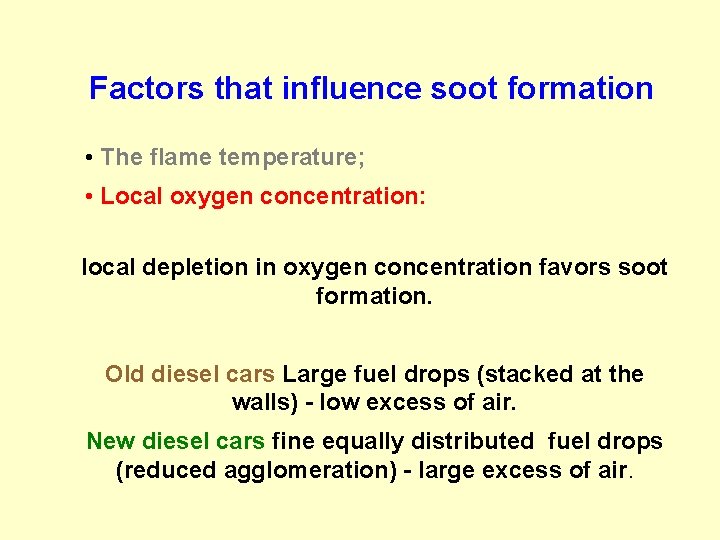
Factors that influence soot formation • The flame temperature; • Local oxygen concentration: local depletion in oxygen concentration favors soot formation. Old diesel cars Large fuel drops (stacked at the walls) - low excess of air. New diesel cars fine equally distributed fuel drops (reduced agglomeration) - large excess of air.
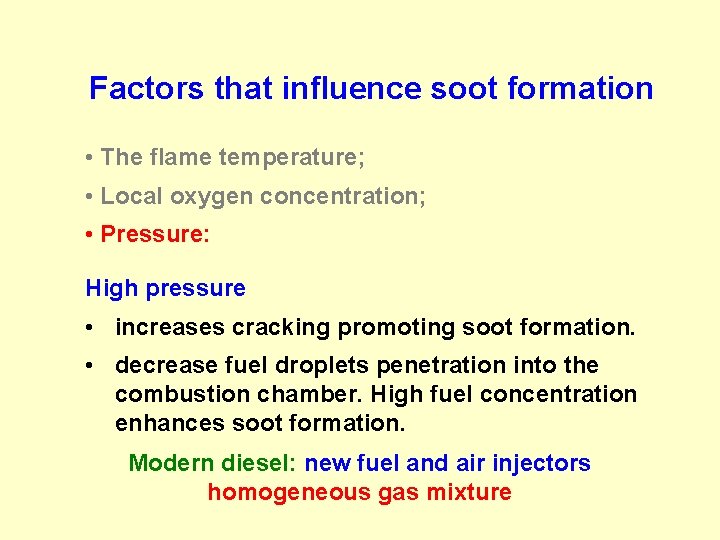
Factors that influence soot formation • The flame temperature; • Local oxygen concentration; • Pressure: High pressure • increases cracking promoting soot formation. • decrease fuel droplets penetration into the combustion chamber. High fuel concentration enhances soot formation. Modern diesel: new fuel and air injectors homogeneous gas mixture
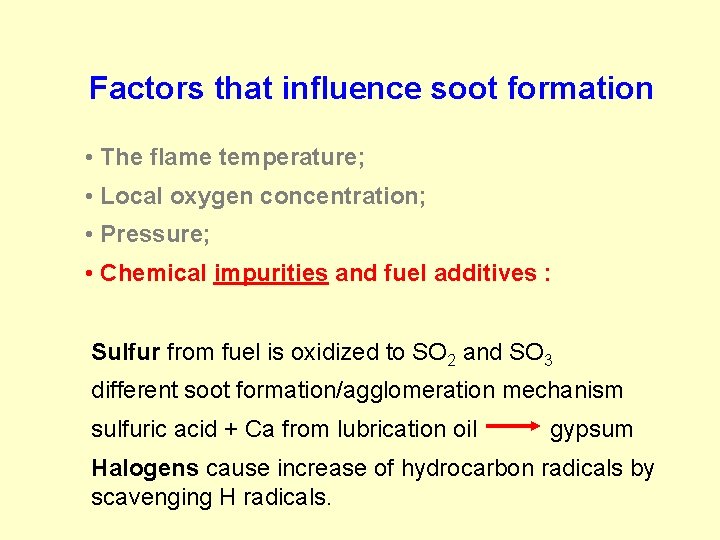
Factors that influence soot formation • The flame temperature; • Local oxygen concentration; • Pressure; • Chemical impurities and fuel additives : Sulfur from fuel is oxidized to SO 2 and SO 3 different soot formation/agglomeration mechanism sulfuric acid + Ca from lubrication oil gypsum Halogens cause increase of hydrocarbon radicals by scavenging H radicals.
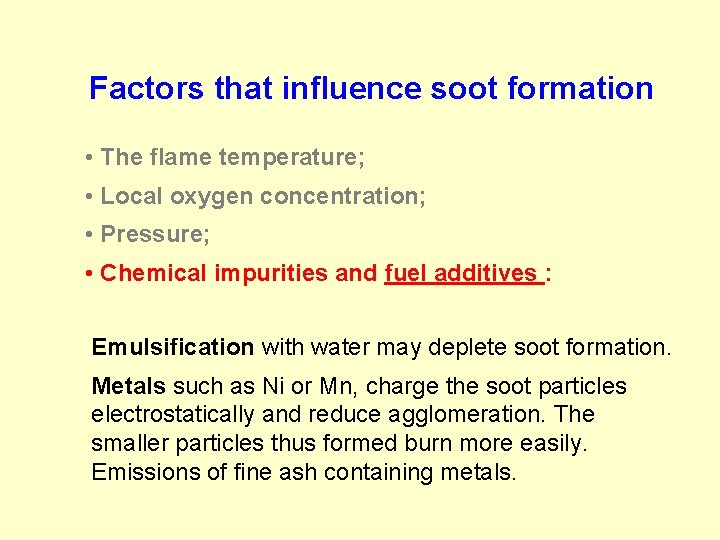
Factors that influence soot formation • The flame temperature; • Local oxygen concentration; • Pressure; • Chemical impurities and fuel additives : Emulsification with water may deplete soot formation. Metals such as Ni or Mn, charge the soot particles electrostatically and reduce agglomeration. The smaller particles thus formed burn more easily. Emissions of fine ash containing metals.
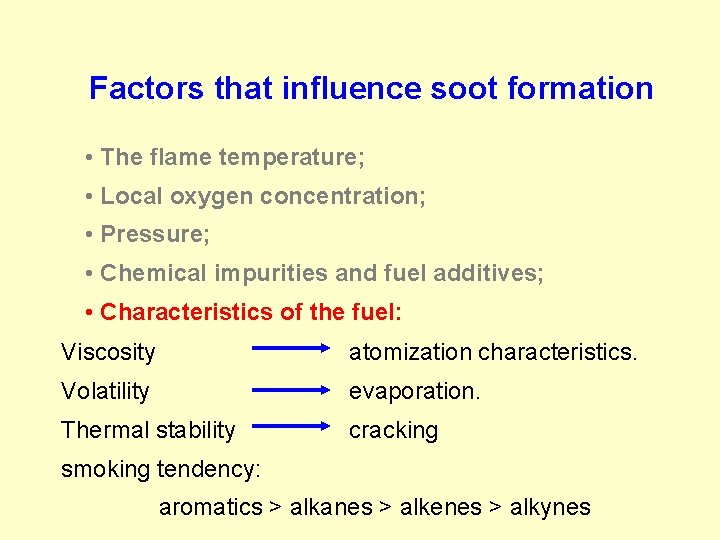
Factors that influence soot formation • The flame temperature; • Local oxygen concentration; • Pressure; • Chemical impurities and fuel additives; • Characteristics of the fuel: Viscosity atomization characteristics. Volatility evaporation. Thermal stability cracking smoking tendency: aromatics > alkanes > alkenes > alkynes
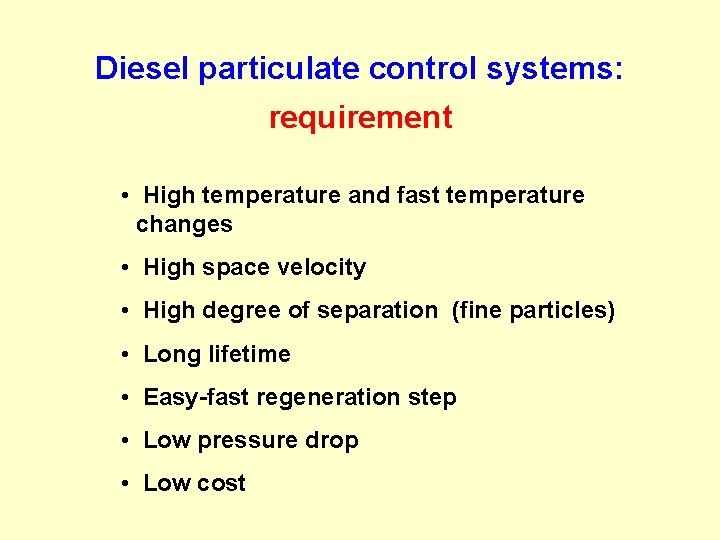
Diesel particulate control systems: requirement • High temperature and fast temperature changes • High space velocity • High degree of separation (fine particles) • Long lifetime • Easy-fast regeneration step • Low pressure drop • Low cost
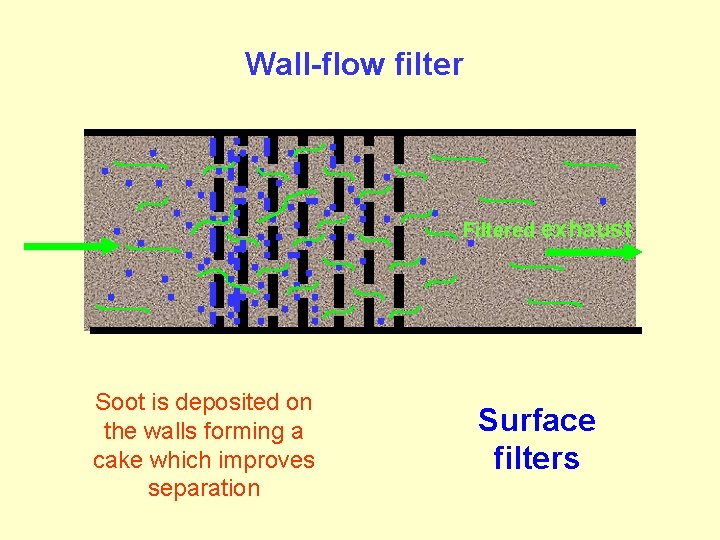
Wall-flow filter Filtered exhaust Soot is deposited on the walls forming a cake which improves separation Surface filters
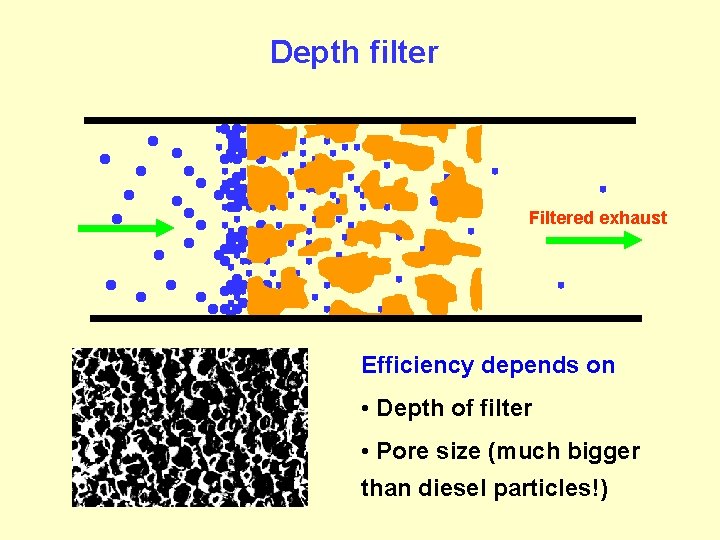
Depth filter Filtered exhaust Efficiency depends on • Depth of filter • Pore size (much bigger than diesel particles!)
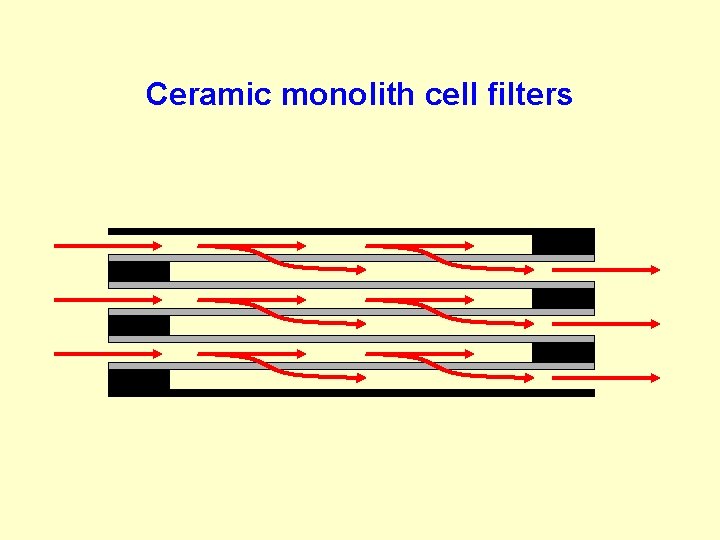
Ceramic monolith cell filters
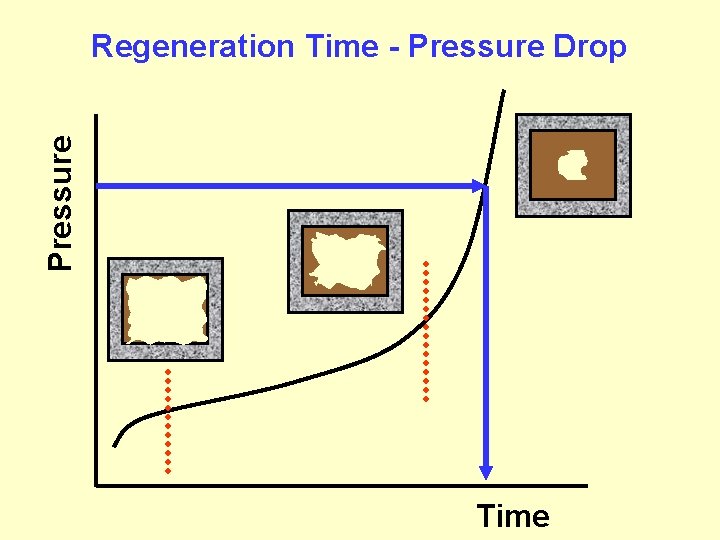
Pressure Regeneration Time - Pressure Drop Time
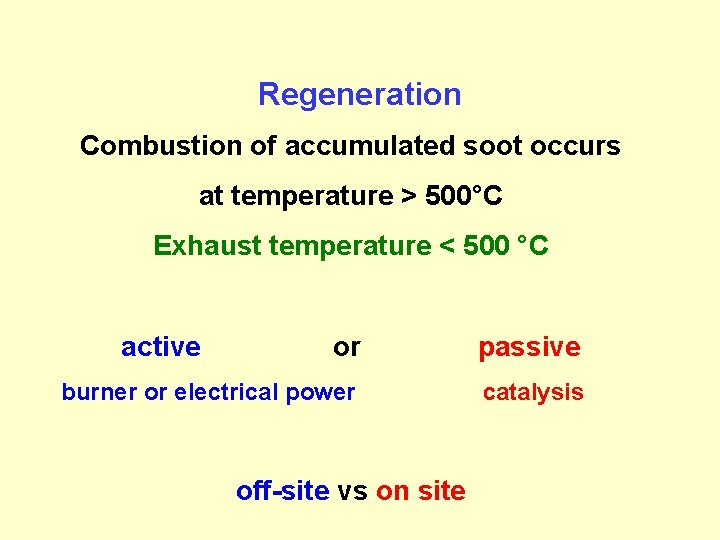
Regeneration Combustion of accumulated soot occurs at temperature > 500°C Exhaust temperature < 500 °C active or passive burner or electrical power catalysis off-site vs on site
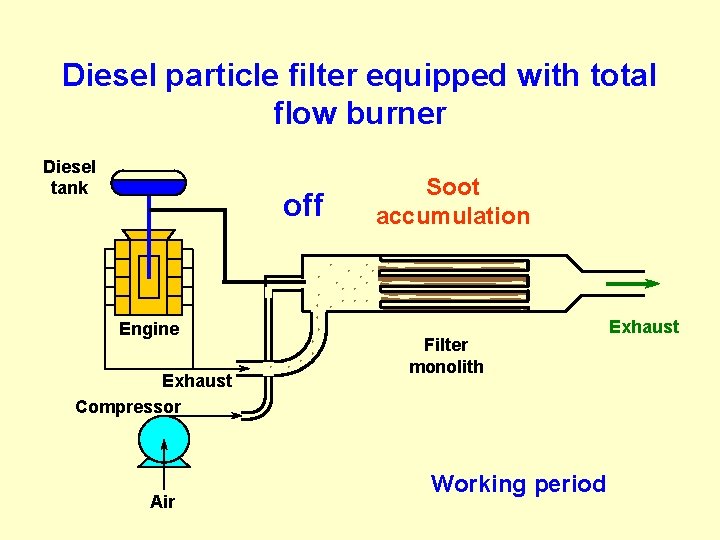
Diesel particle filter equipped with total flow burner Diesel tank off Engine Exhaust Compressor Air Soot accumulation Filter monolith Working period Exhaust
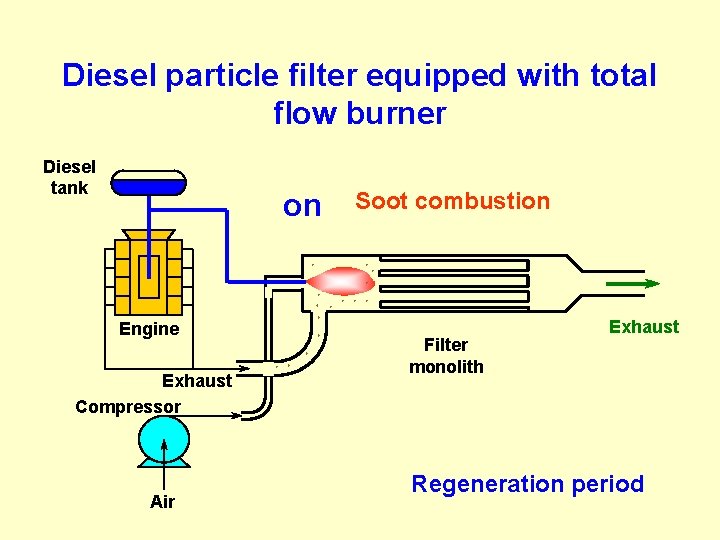
Diesel particle filter equipped with total flow burner Diesel tank on Engine Exhaust Compressor Air Soot combustion Filter monolith Exhaust Regeneration period
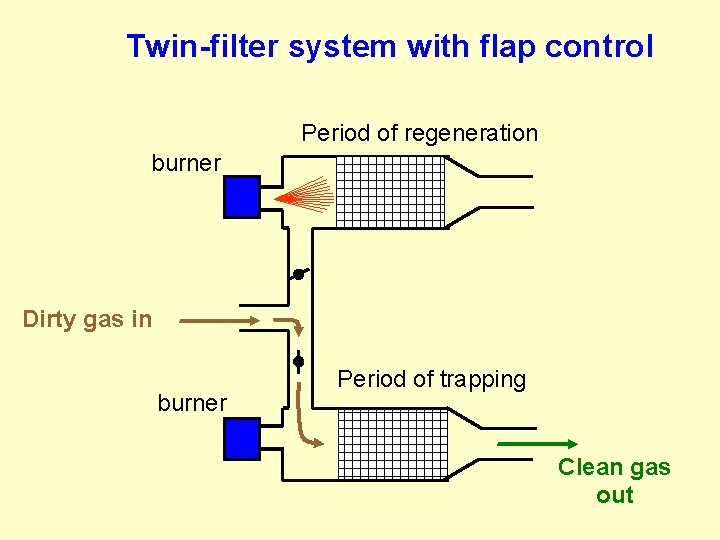
Twin-filter system with flap control Period of regeneration burner Dirty gas in burner Period of trapping Clean gas out
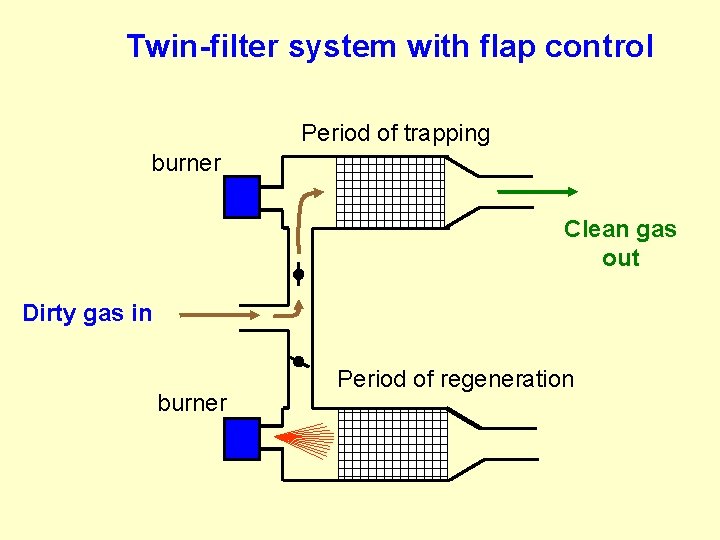
Twin-filter system with flap control Period of trapping burner Clean gas out Dirty gas in burner Period of regeneration
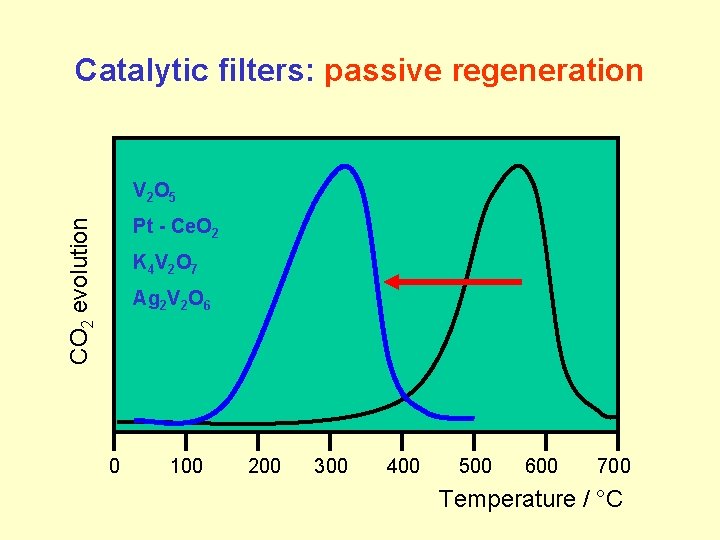
Catalytic filters: passive regeneration V 2 O 5 CO 2 evolution Pt - Ce. O 2 K 4 V 2 O 7 Ag 2 V 2 O 6 0 100 200 300 400 500 600 700 Temperature / °C
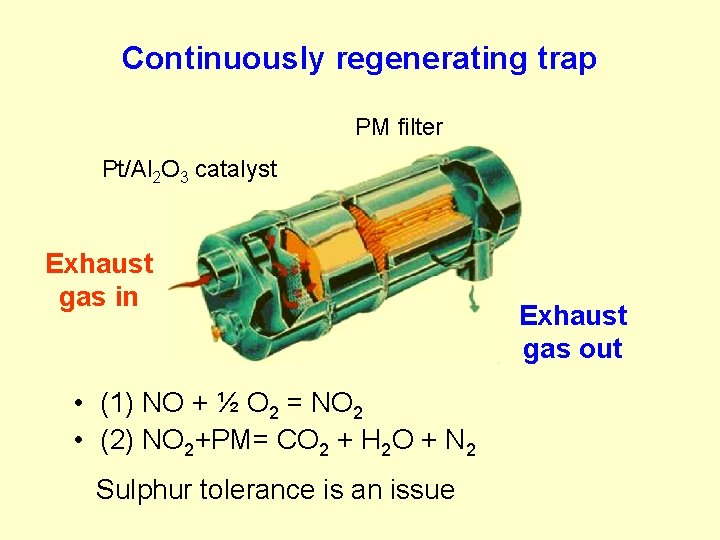
Continuously regenerating trap PM filter Pt/Al 2 O 3 catalyst Exhaust gas in • (1) NO + ½ O 2 = NO 2 • (2) NO 2+PM= CO 2 + H 2 O + N 2 Sulphur tolerance is an issue Exhaust gas out
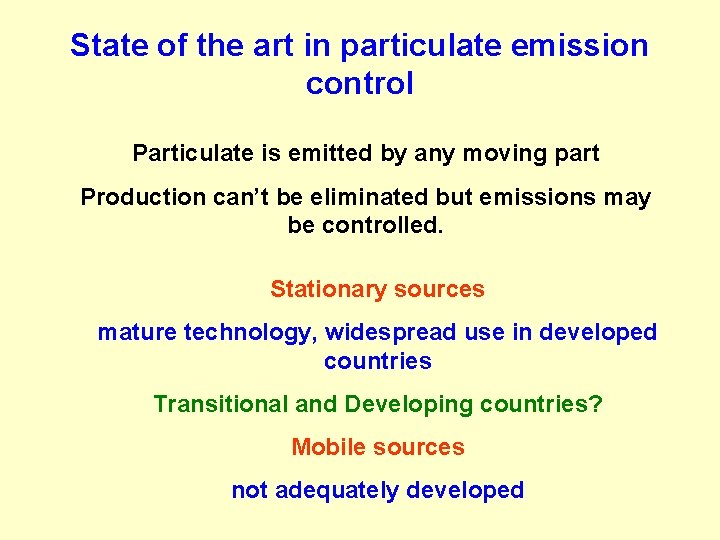
State of the art in particulate emission control Particulate is emitted by any moving part Production can’t be eliminated but emissions may be controlled. Stationary sources mature technology, widespread use in developed countries Transitional and Developing countries? Mobile sources not adequately developed
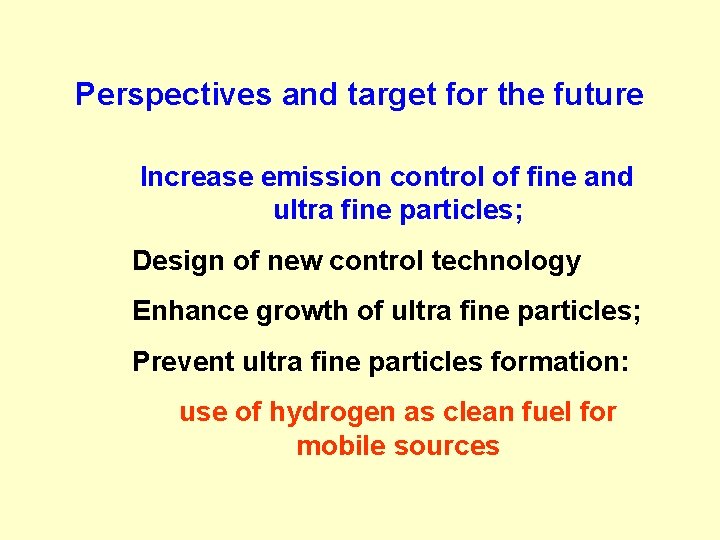
Perspectives and target for the future Increase emission control of fine and ultra fine particles; Design of new control technology Enhance growth of ultra fine particles; Prevent ultra fine particles formation: use of hydrogen as clean fuel for mobile sources
Wesam al madhoun
Control system
Emission control system repair
Particulate processing adalah
Particulate model of matter
Particulate contamination in infusions
Die blanks ceramics
Confined space dredging
Particle theory of matter
God of radiation
Which are the main group elements
Who was mendal
Radioactive examples
Beta particle symbol
Positron emission
Einstein equation for photoelectric emission is
Microwave amplification by stimulated emission of radiation
Metastable state in laser
Stimulated emission
Stimulated emission
Bandplan tingkat siaga pada band vhf
Bread toasting: gas formation color change light emission
Partial periodic table
Positron emission tomography
Advantages of flame emission spectroscopy
Uranium 238 alpha decay equation
Fluorescence spectroscopy - ppt
Atomic emmision spectrum
Atomic emission spectroscopy principle
Difference between absorption and emission spectrum
Emission vs ejaculation
Miller zoo emission
Absorption vs emission
Atomic emission spectra and the quantum mechanical model
Lepton number table
Is code 800
Photo emission microscopy failure analysis
Plastic oled
Atomic emission spectra periodic table
Vmware latf
Photometry
Emission vs ejaculation
Uranium 238 decay equation
Acoustic emission testing applications
Atomic emission spectra and the quantum mechanical model
Positron emission tomography
Positron emission tomography
Complexe g emission
Matter and materials grade 12
Why are atomic emission spectra discontinuous
Atomic emission spectroscopy lecture notes
Energy emission
Energy emission
Positron emission
The pcv system controls which exhaust emission(s)?
Tga vean
Synthesis
Energy emission