Introduction to Analytical Chemistry CHAPTER 13 POTENTIOMETRY MEASURING
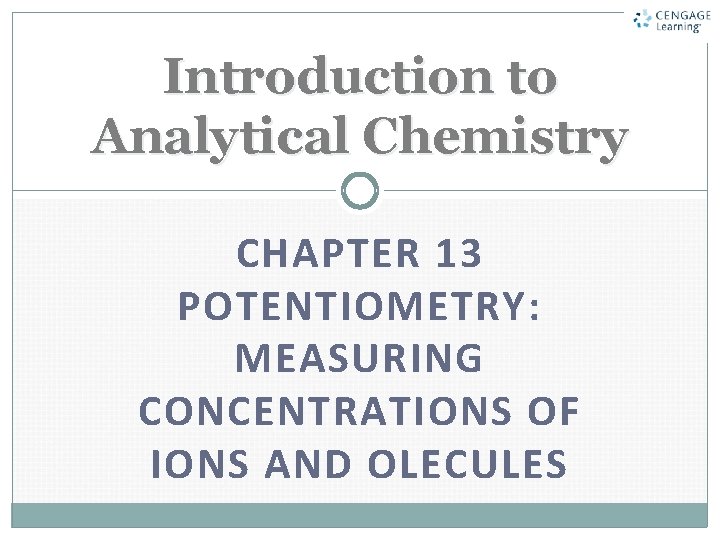
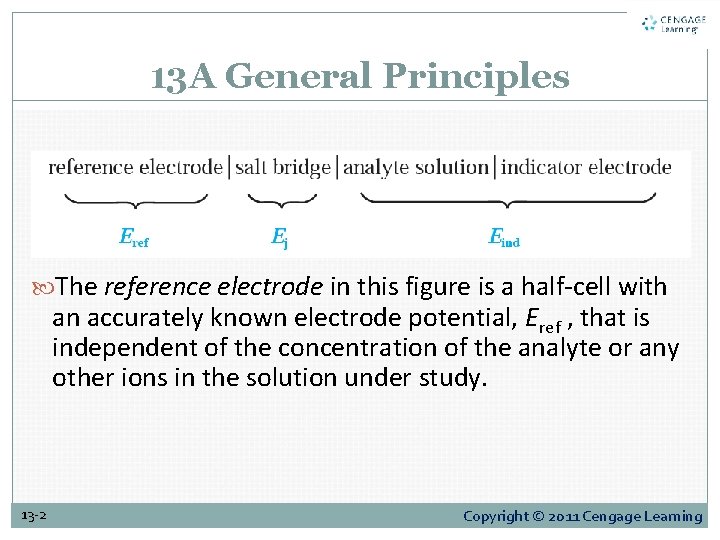
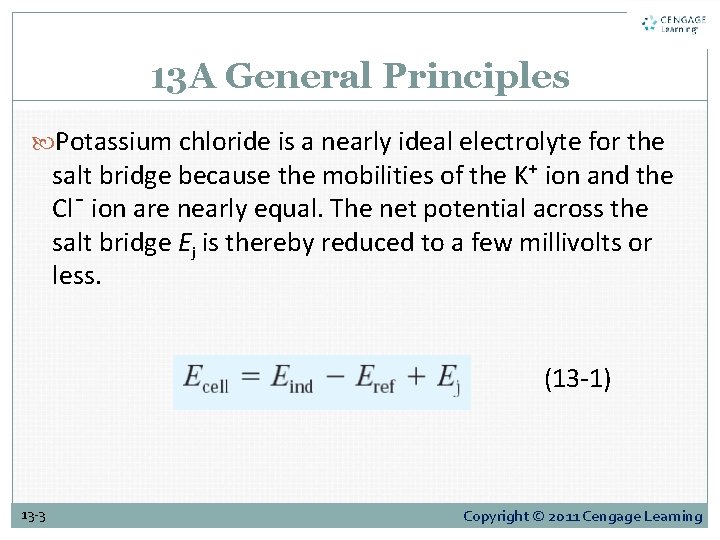
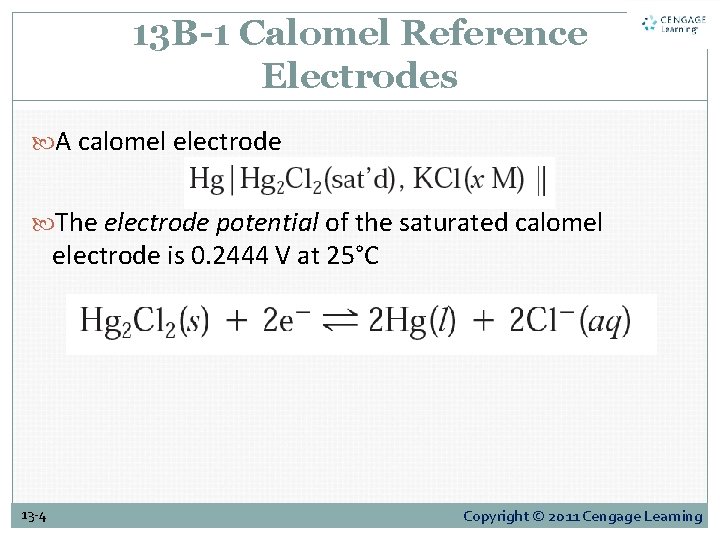
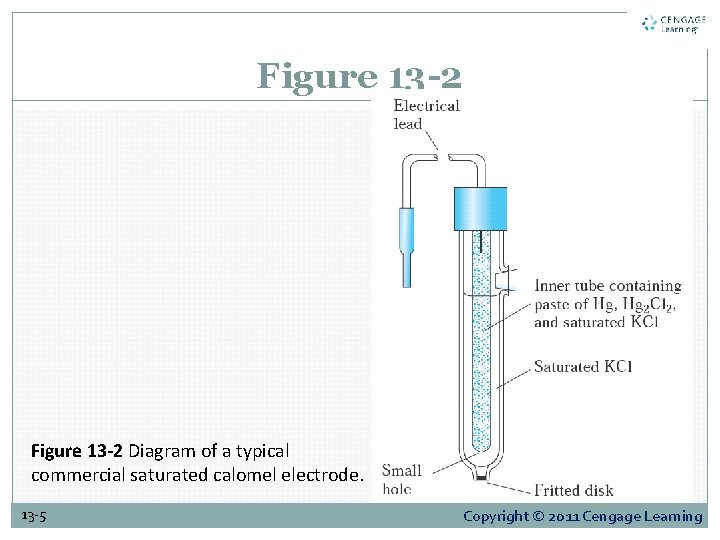
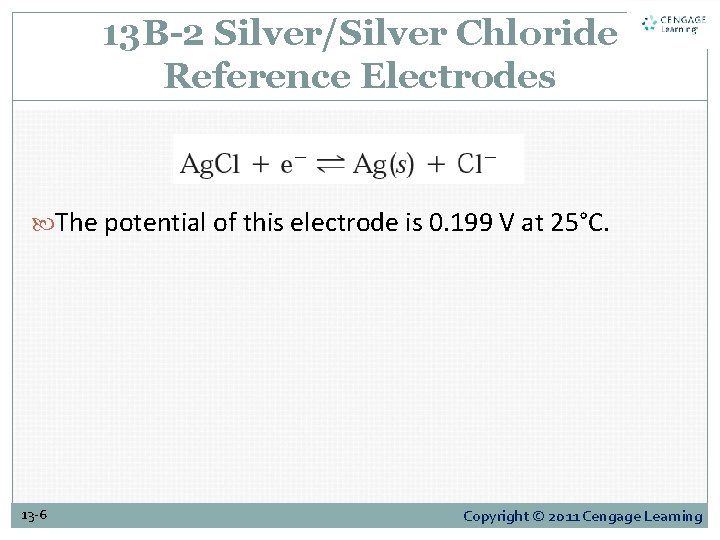
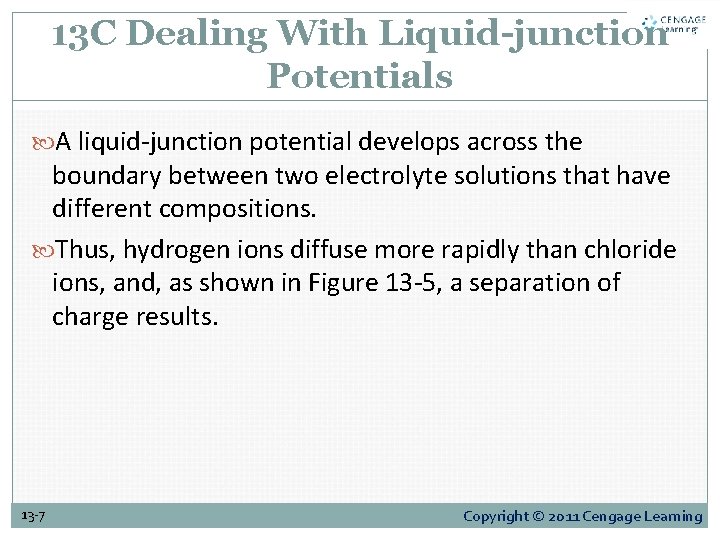
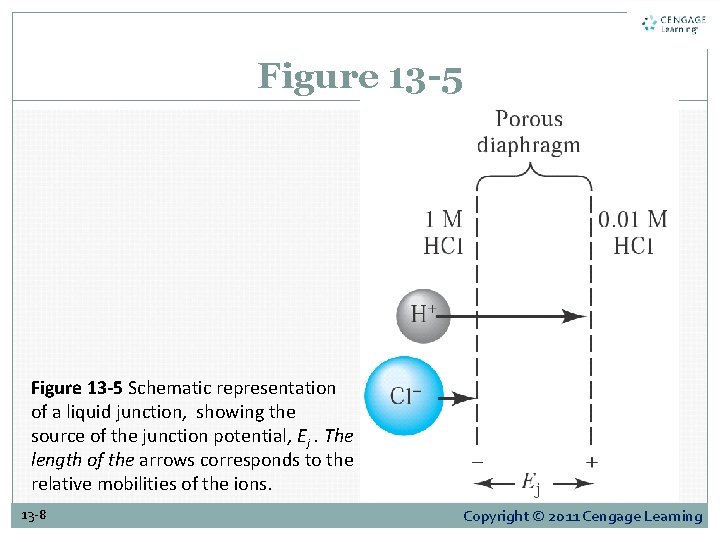
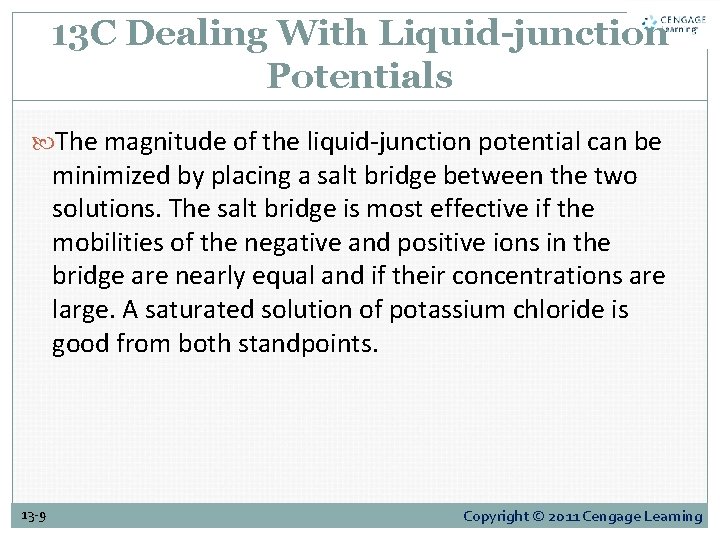
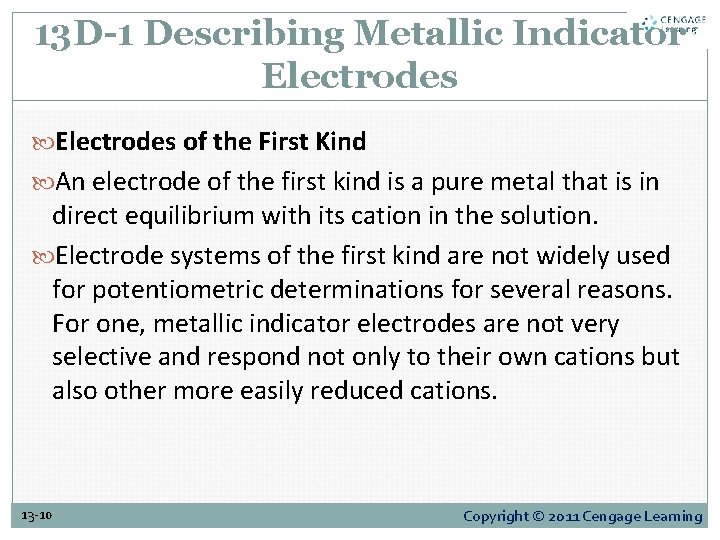
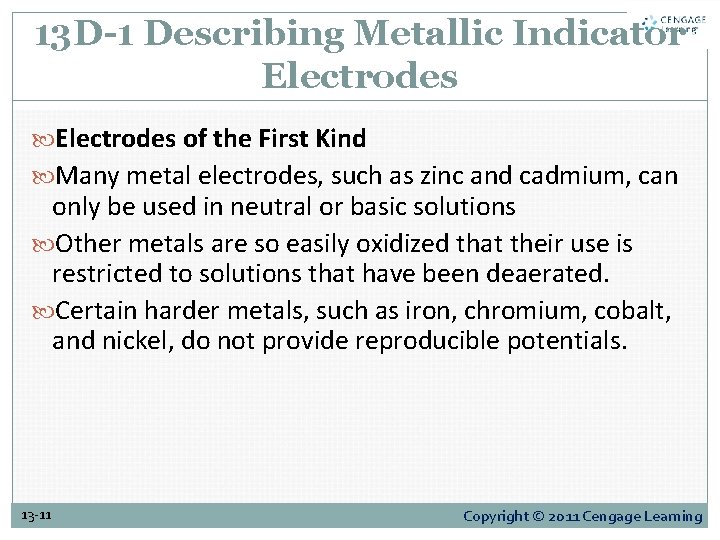
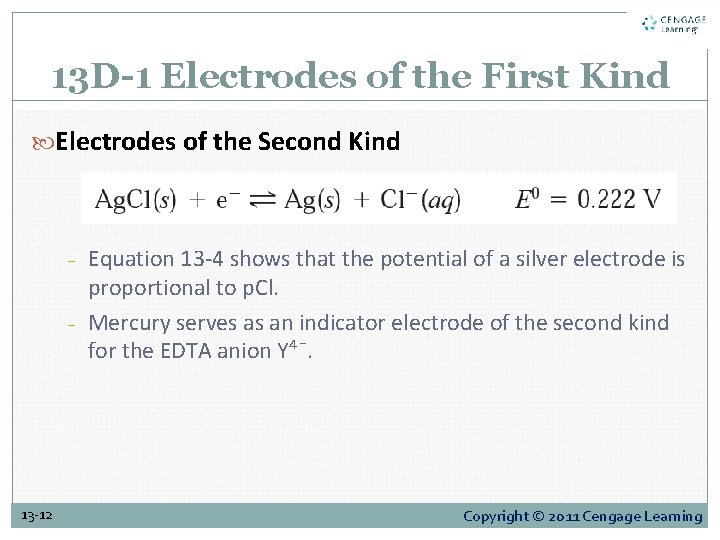
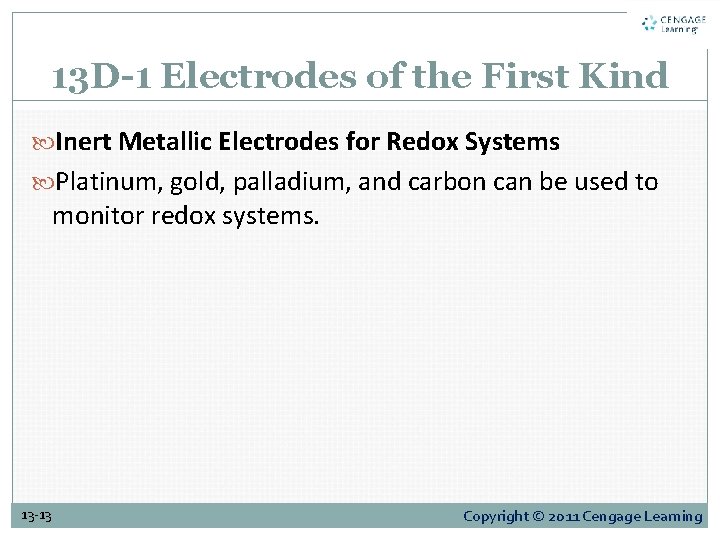
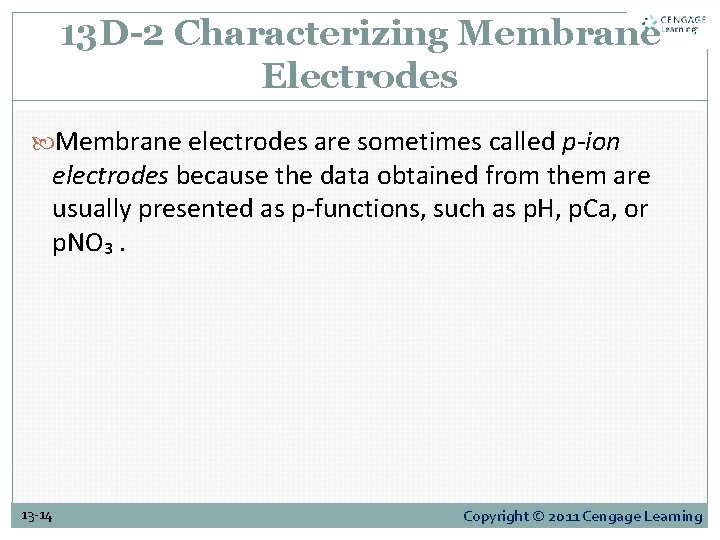
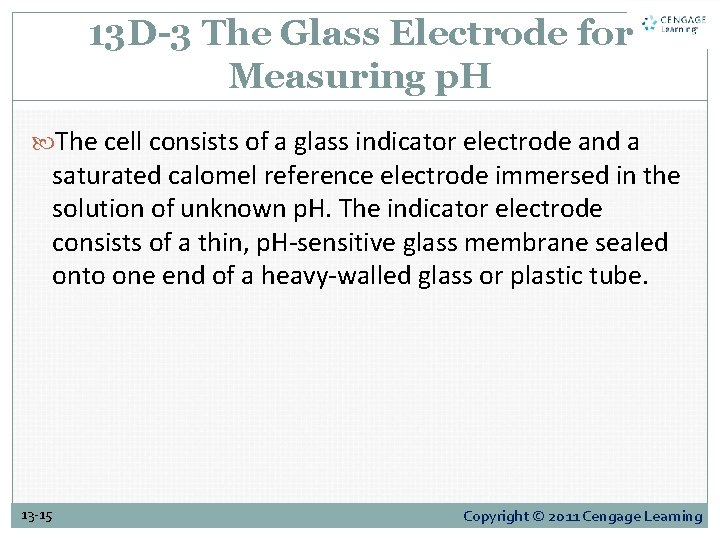
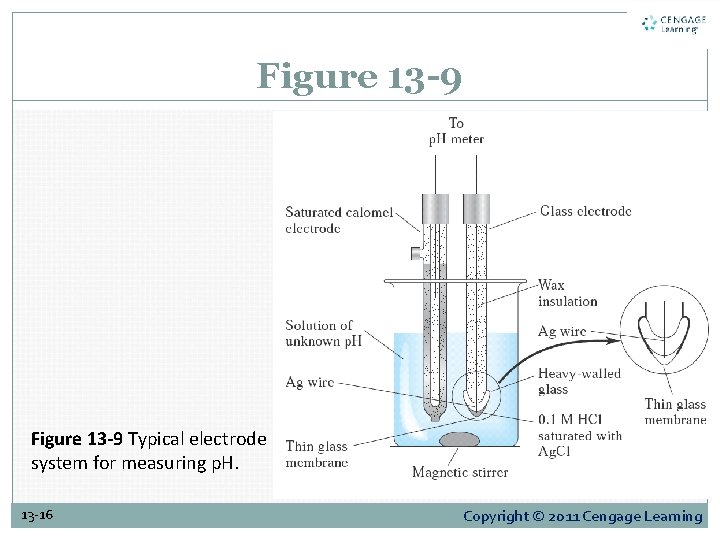
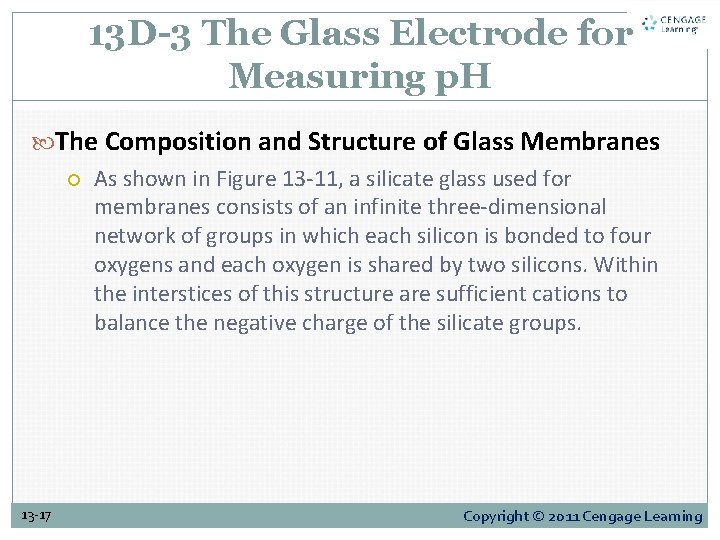
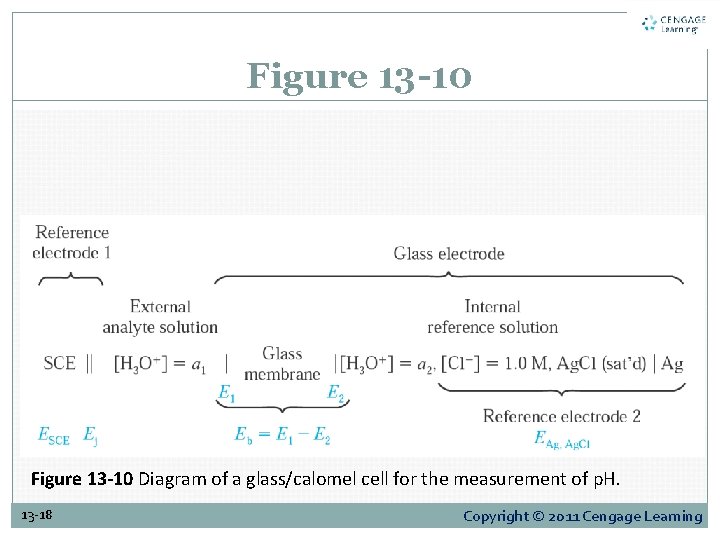
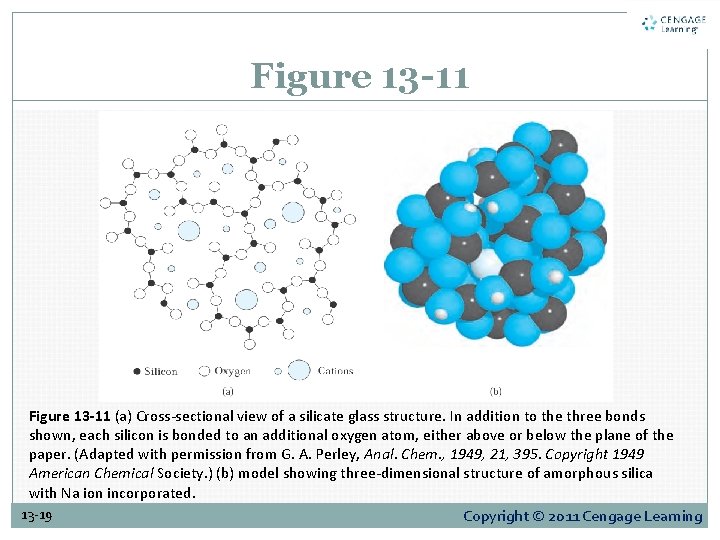
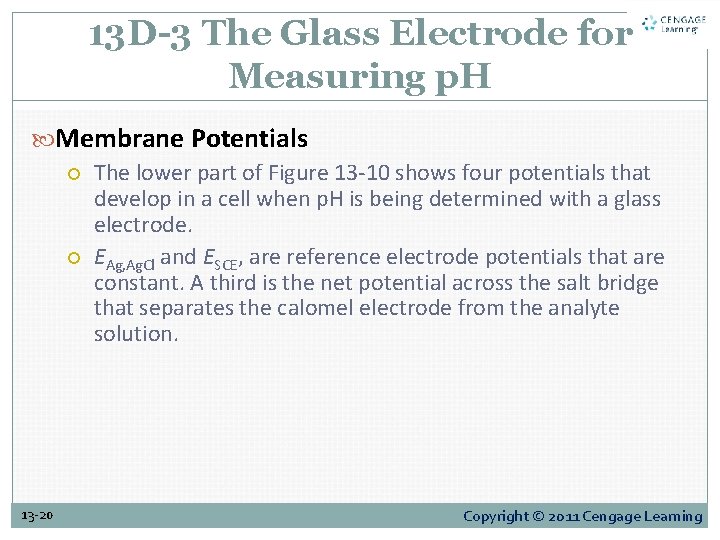
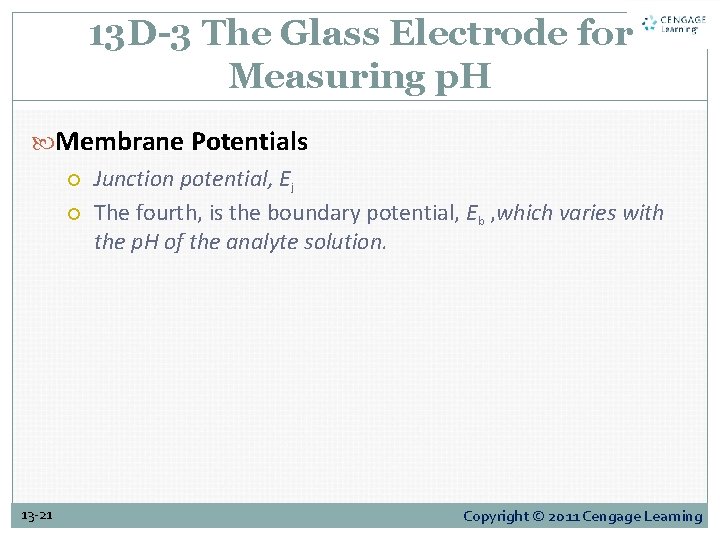
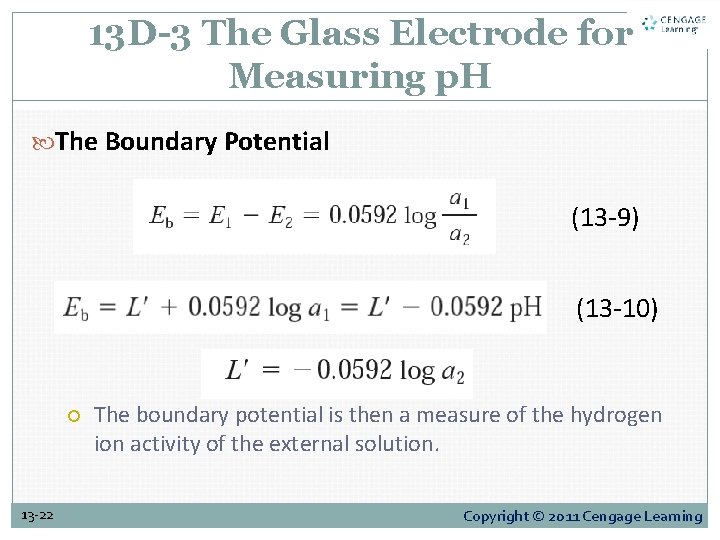
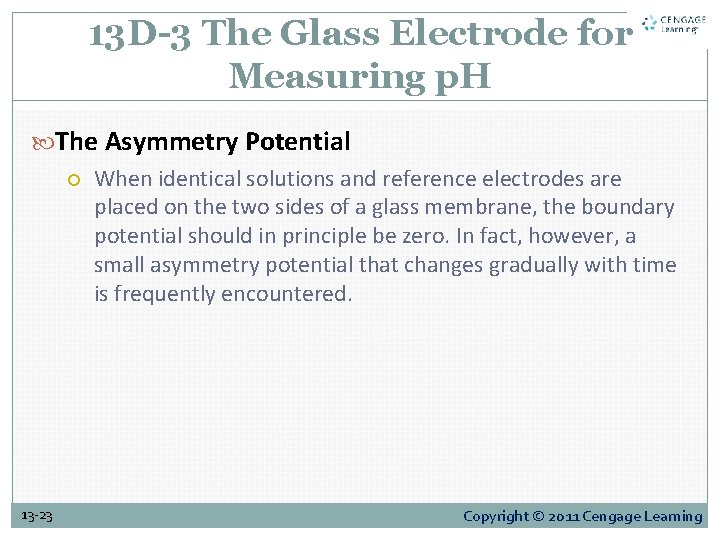
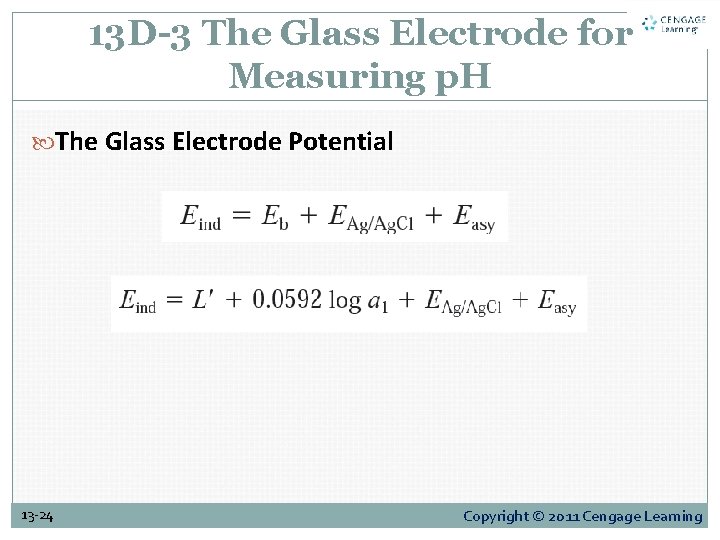
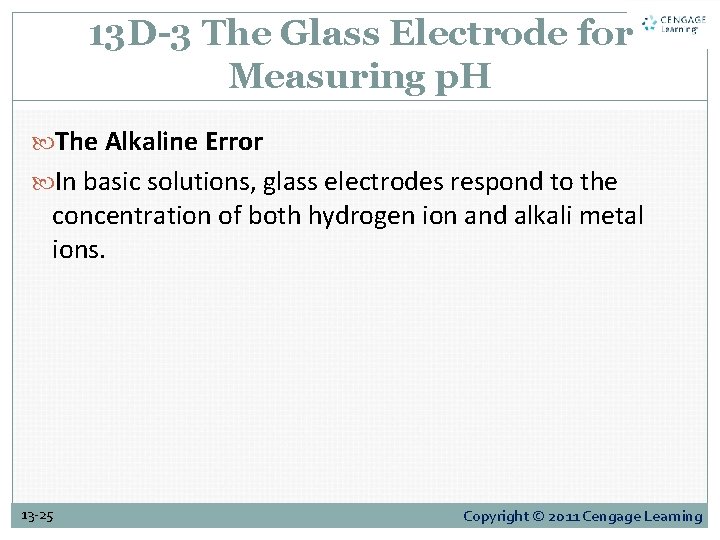
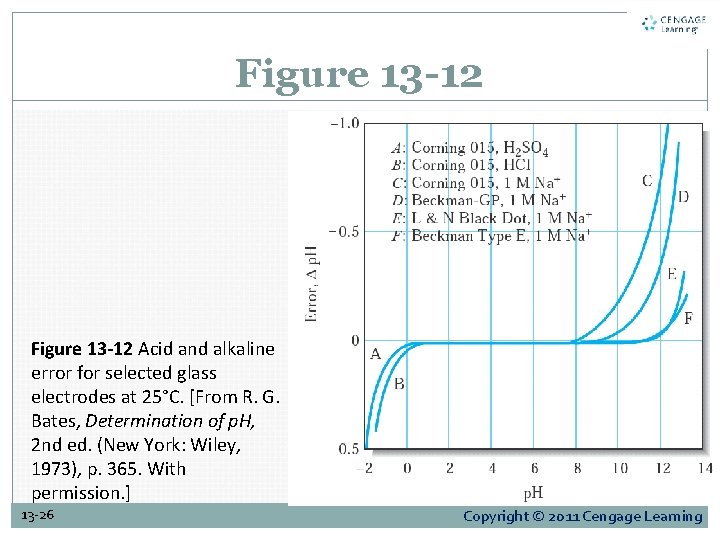
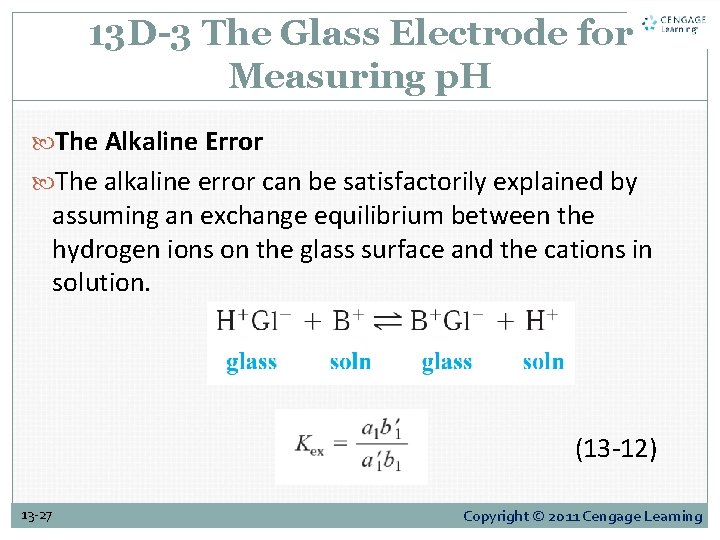
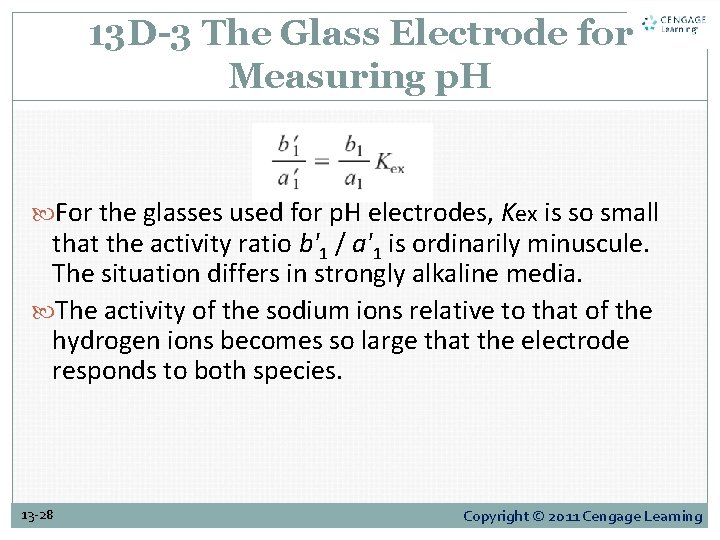
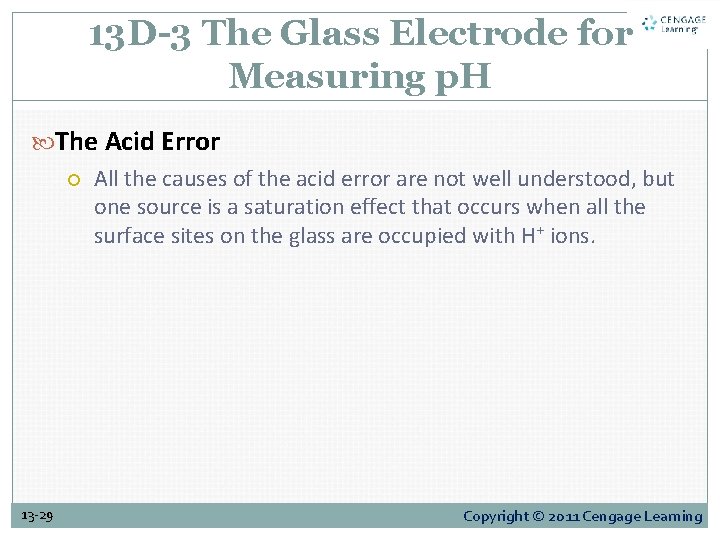
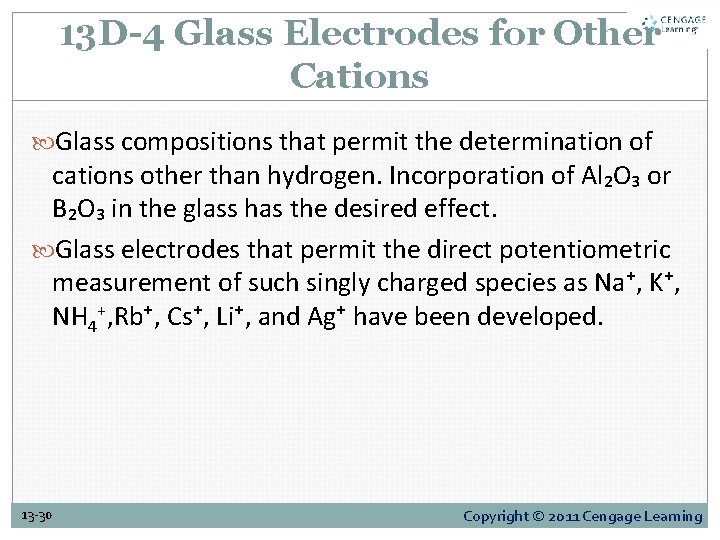
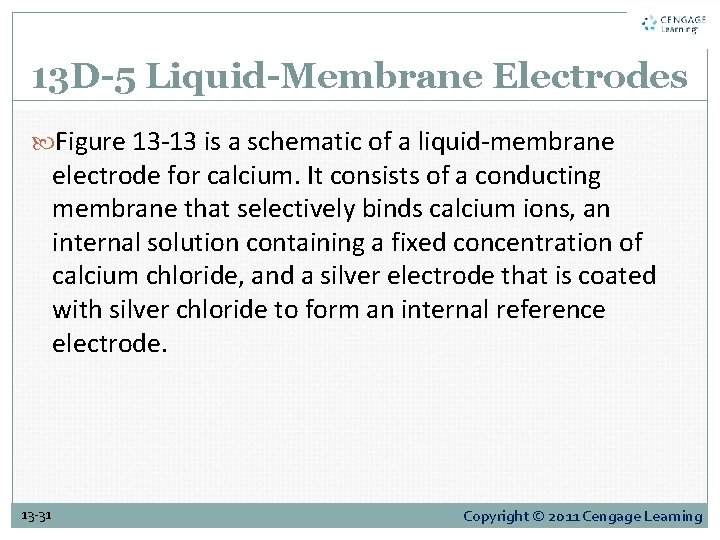
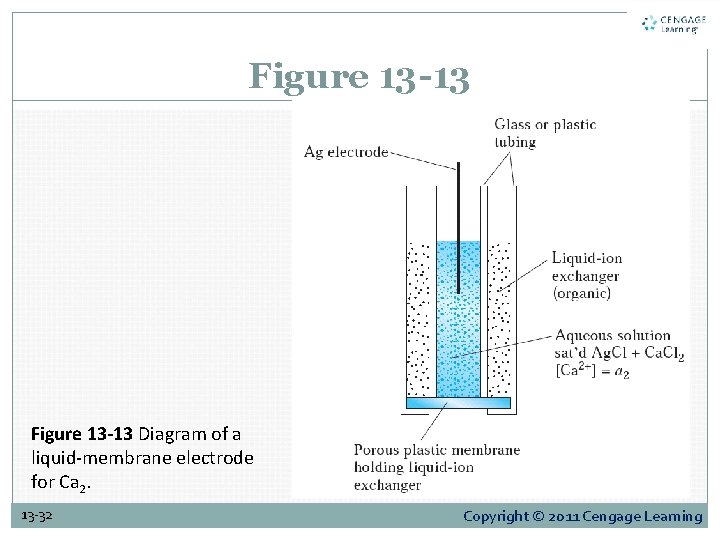
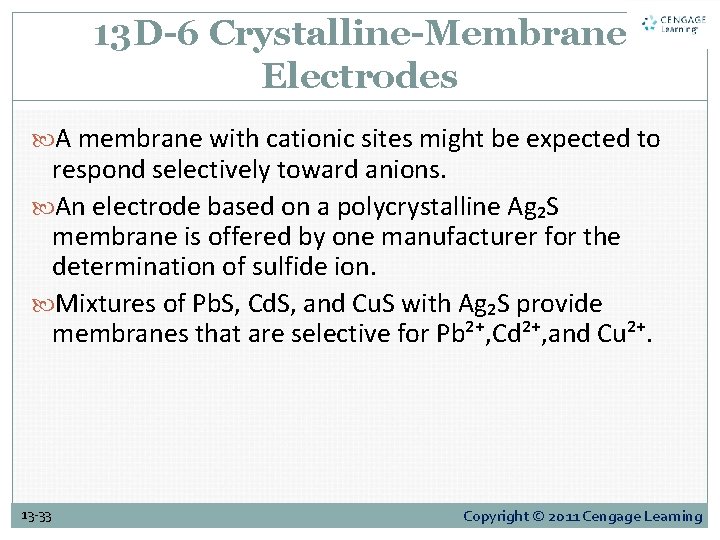
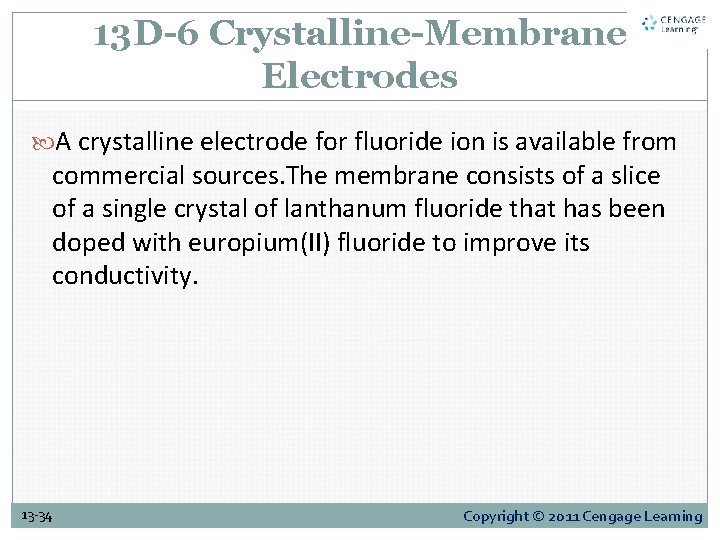
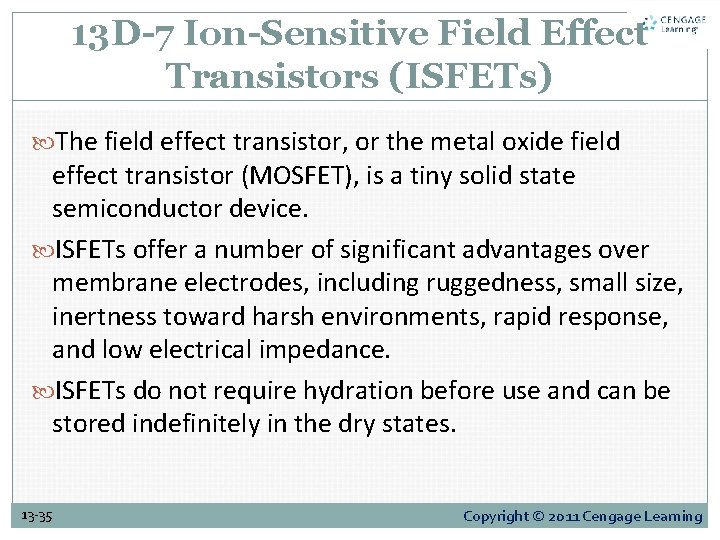
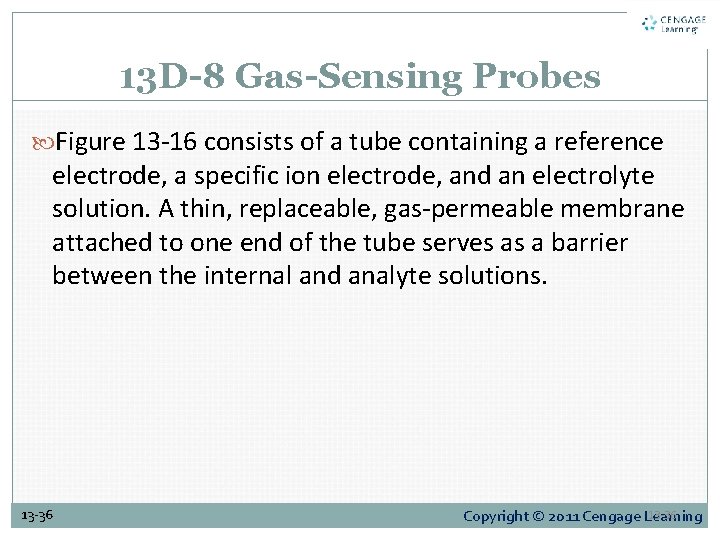
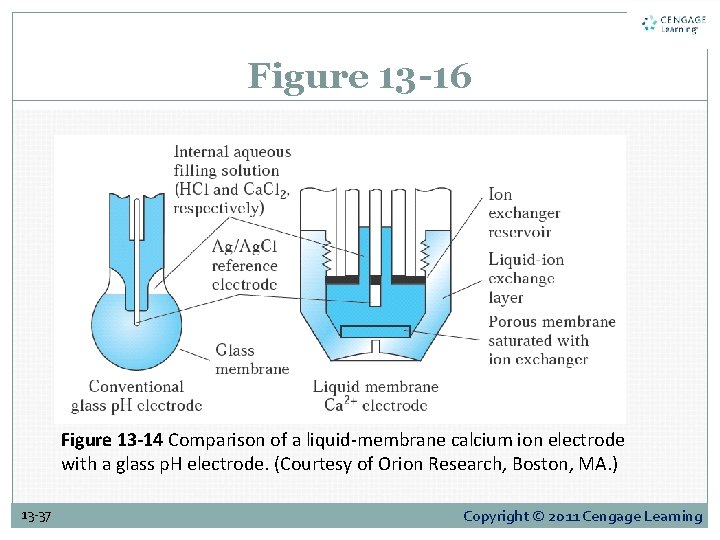
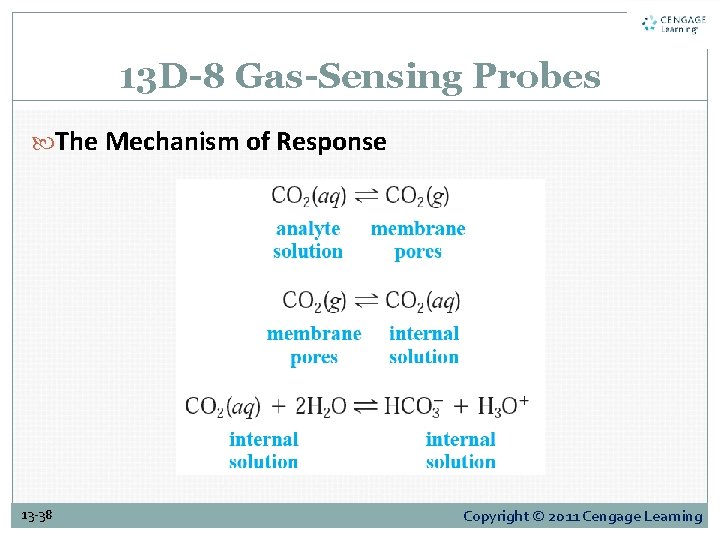
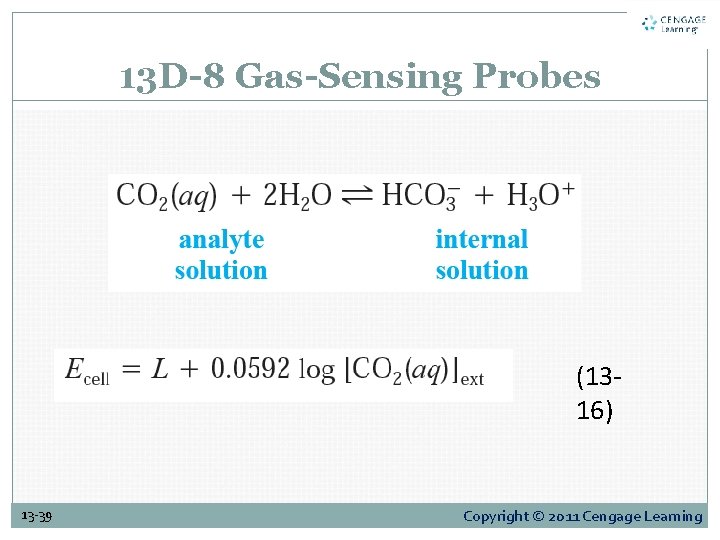
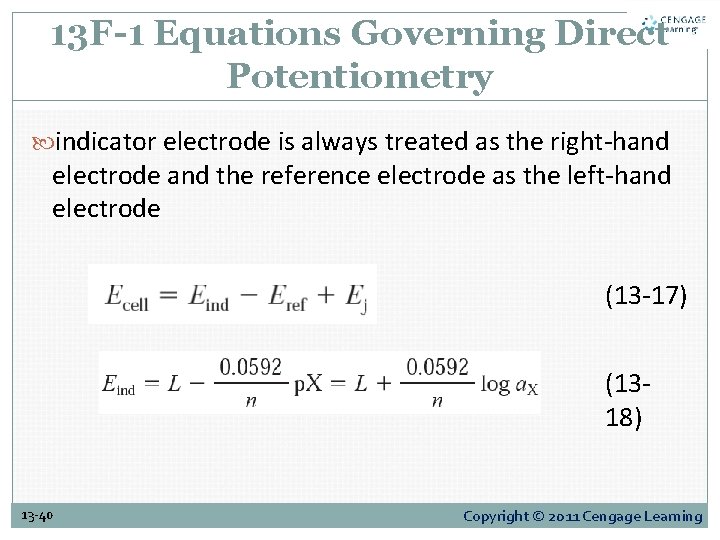
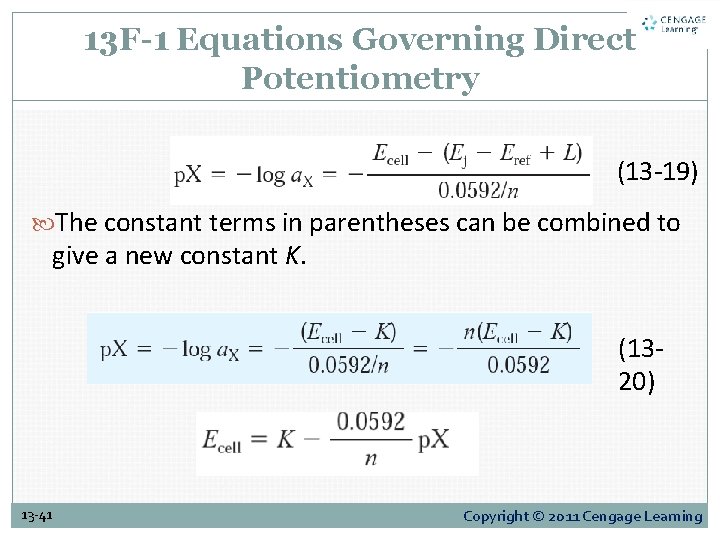
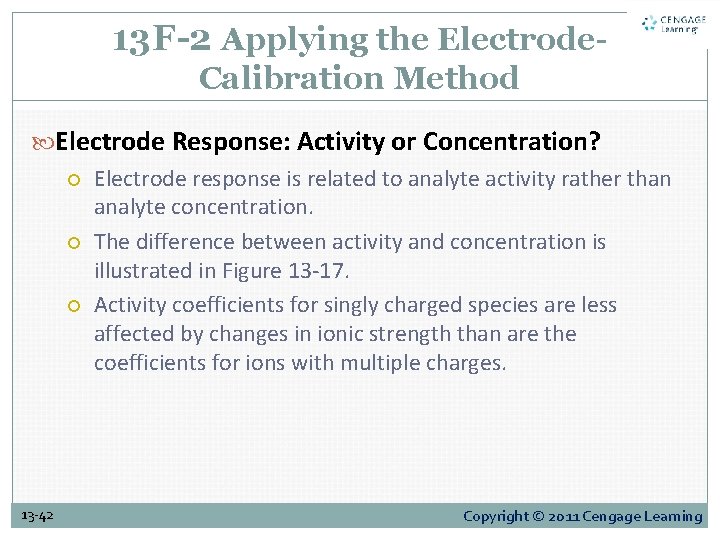
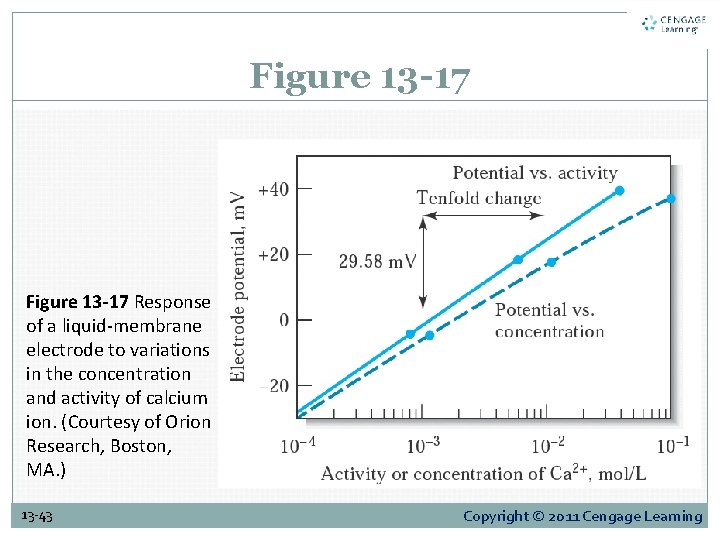
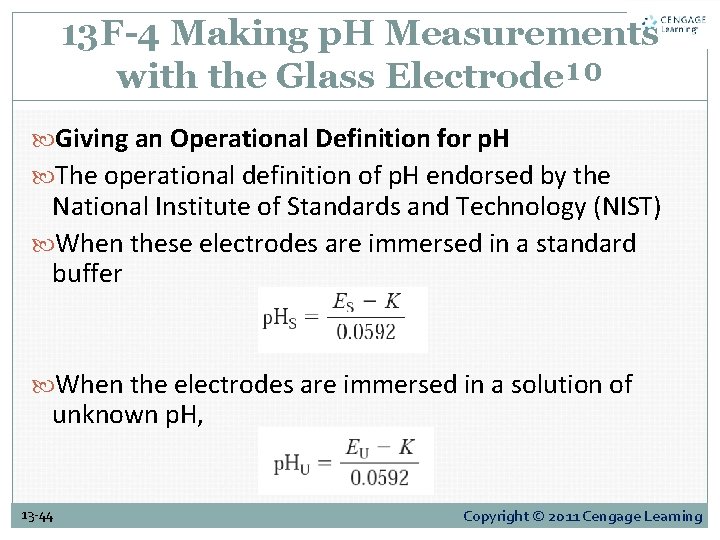
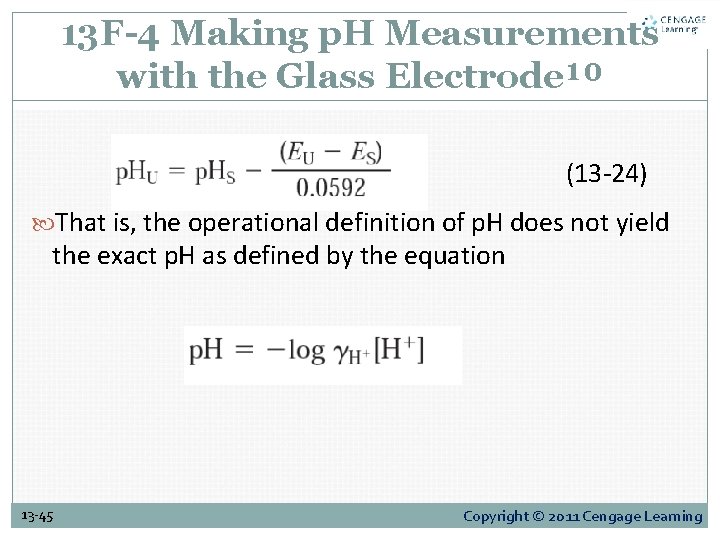
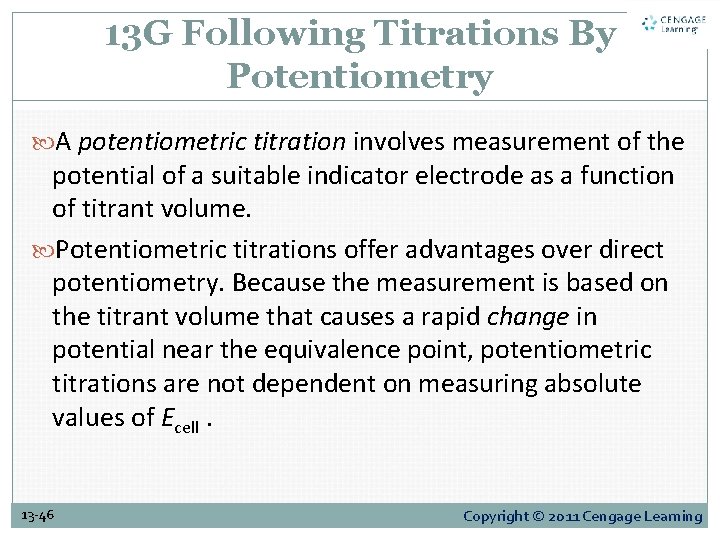
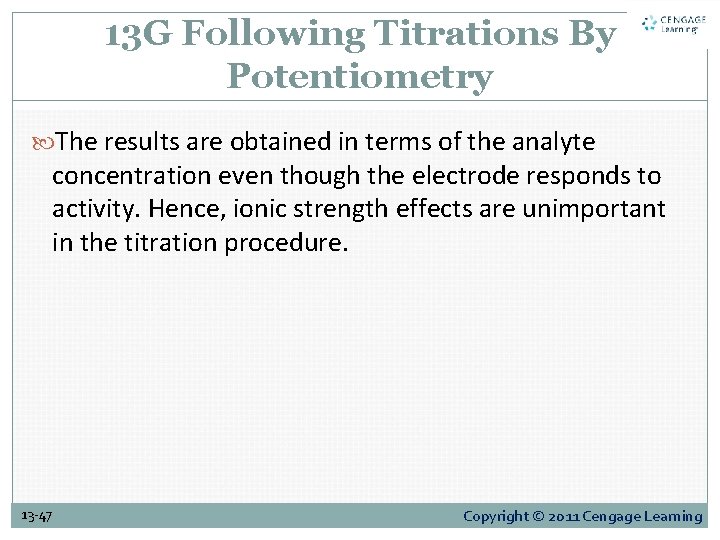
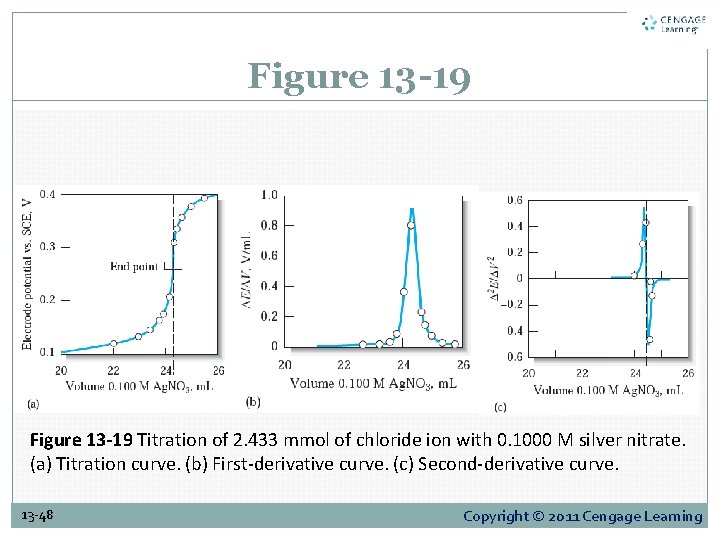
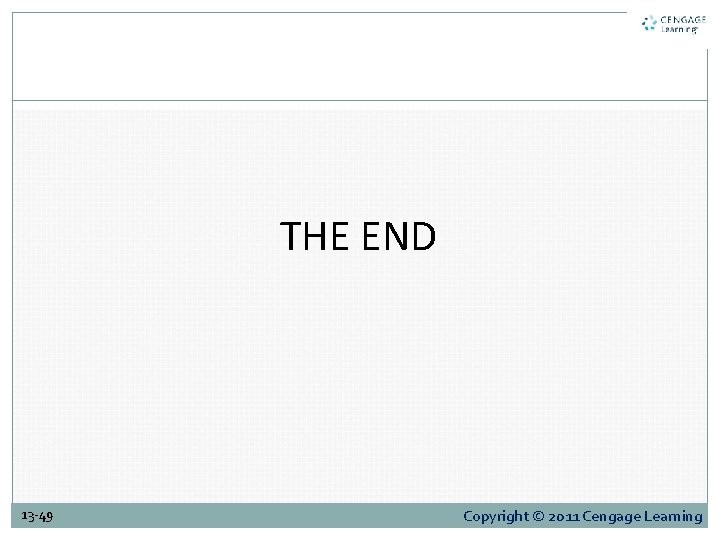
- Slides: 49
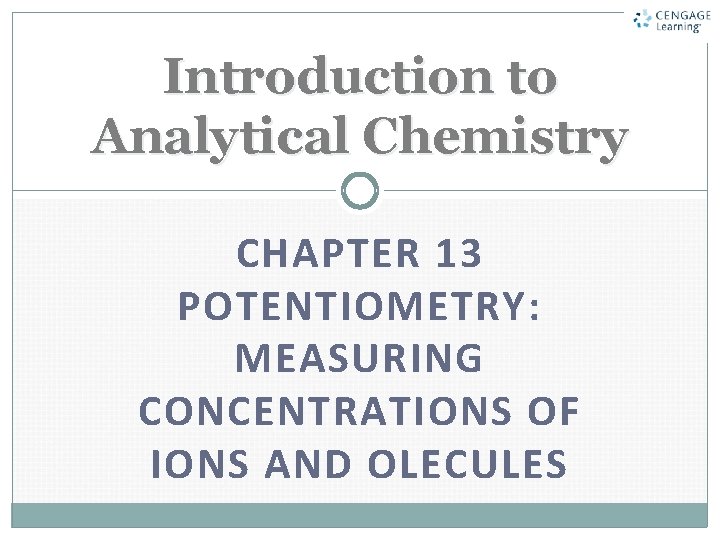
Introduction to Analytical Chemistry CHAPTER 13 POTENTIOMETRY: MEASURING CONCENTRATIONS OF IONS AND OLECULES
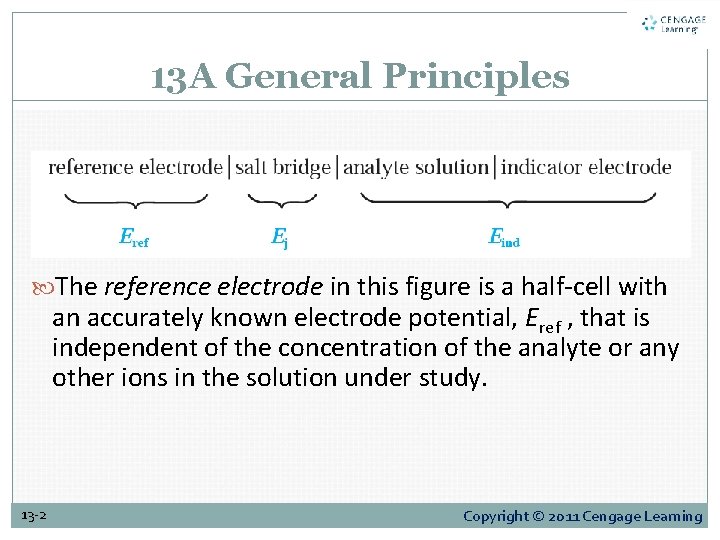
13 A General Principles The reference electrode in this figure is a half-cell with an accurately known electrode potential, Eref , that is independent of the concentration of the analyte or any other ions in the solution under study. 13 -2 Copyright © 2011 Cengage Learning
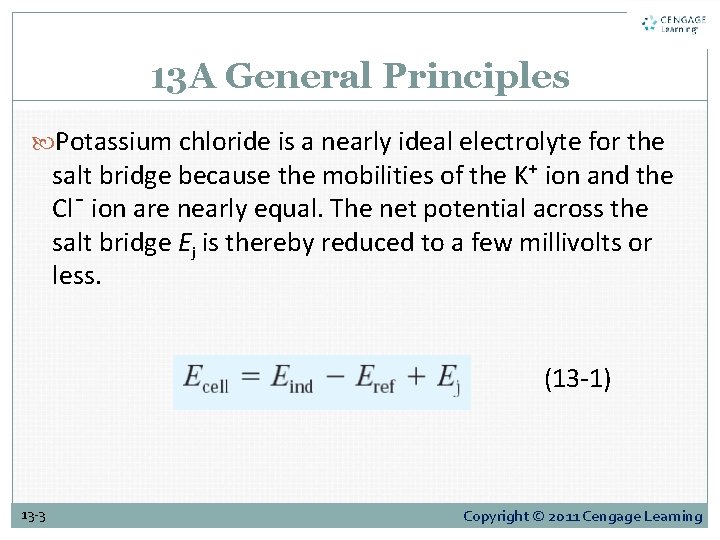
13 A General Principles Potassium chloride is a nearly ideal electrolyte for the salt bridge because the mobilities of the K⁺ ion and the Cl¯ ion are nearly equal. The net potential across the salt bridge Ej is thereby reduced to a few millivolts or less. (13 -1) 13 -3 Copyright © 2011 Cengage Learning
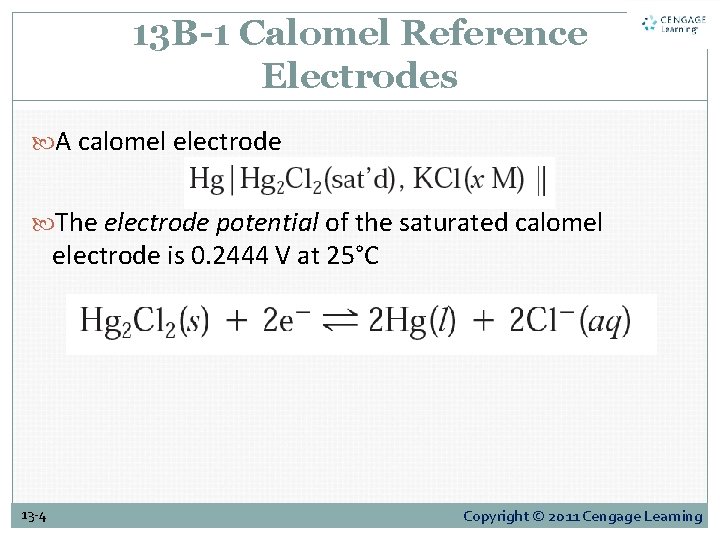
13 B-1 Calomel Reference Electrodes A calomel electrode The electrode potential of the saturated calomel electrode is 0. 2444 V at 25°C 13 -4 Copyright © 2011 Cengage Learning
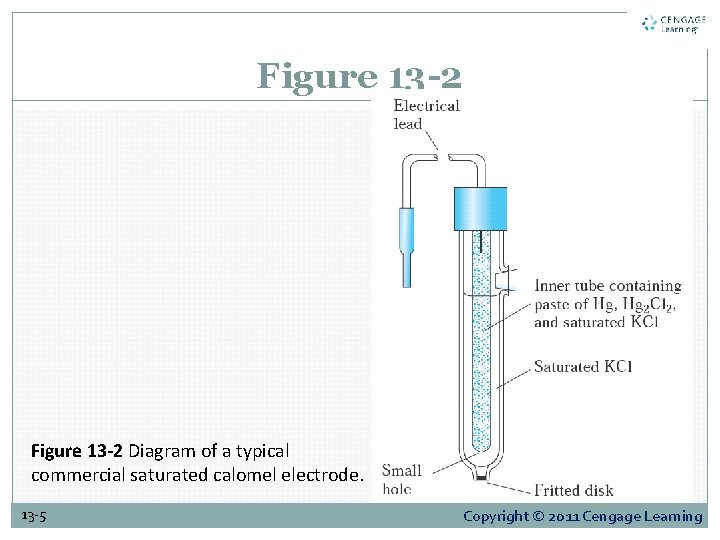
Figure 13 -2 Diagram of a typical commercial saturated calomel electrode. 13 -5 Copyright © 2011 Cengage Learning
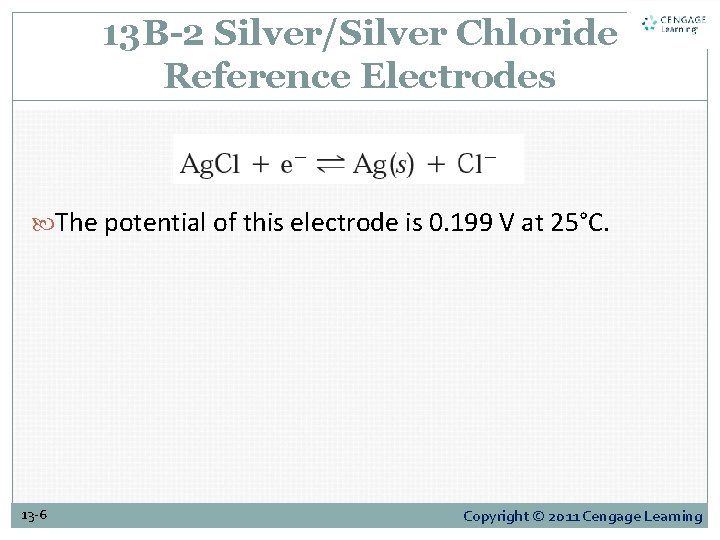
13 B-2 Silver/Silver Chloride Reference Electrodes The potential of this electrode is 0. 199 V at 25°C. 13 -6 Copyright © 2011 Cengage Learning
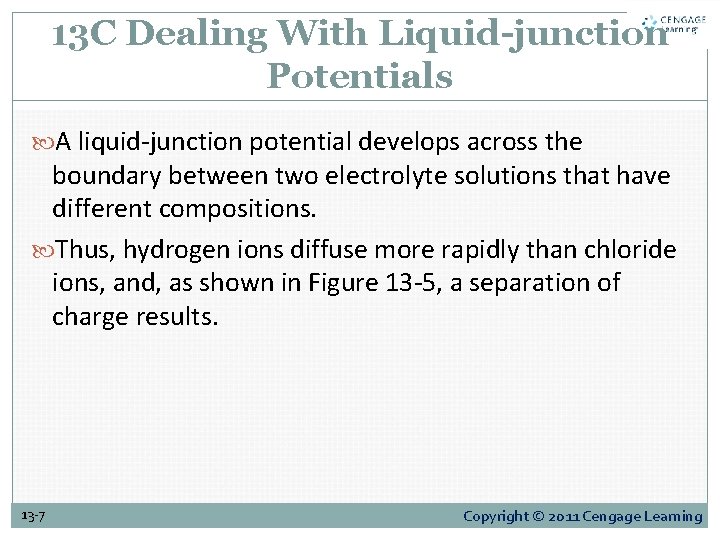
13 C Dealing With Liquid-junction Potentials A liquid-junction potential develops across the boundary between two electrolyte solutions that have different compositions. Thus, hydrogen ions diffuse more rapidly than chloride ions, and, as shown in Figure 13 -5, a separation of charge results. 13 -7 Copyright © 2011 Cengage Learning
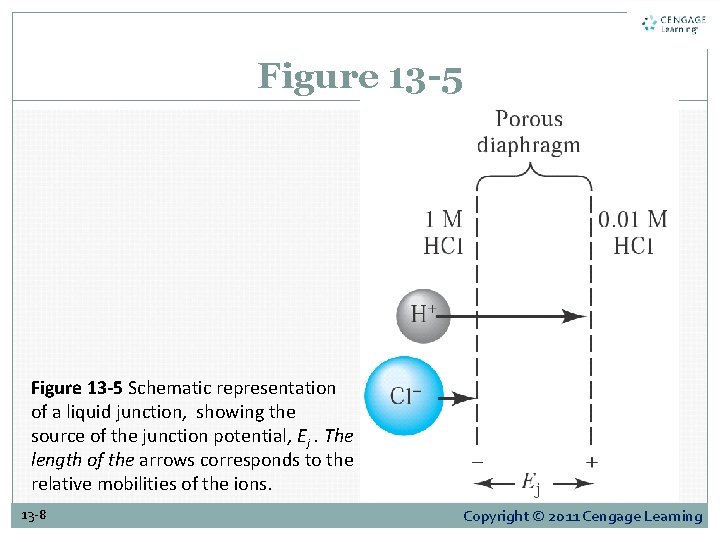
Figure 13 -5 Schematic representation of a liquid junction, showing the source of the junction potential, Ej. The length of the arrows corresponds to the relative mobilities of the ions. 13 -8 Copyright © 2011 Cengage Learning
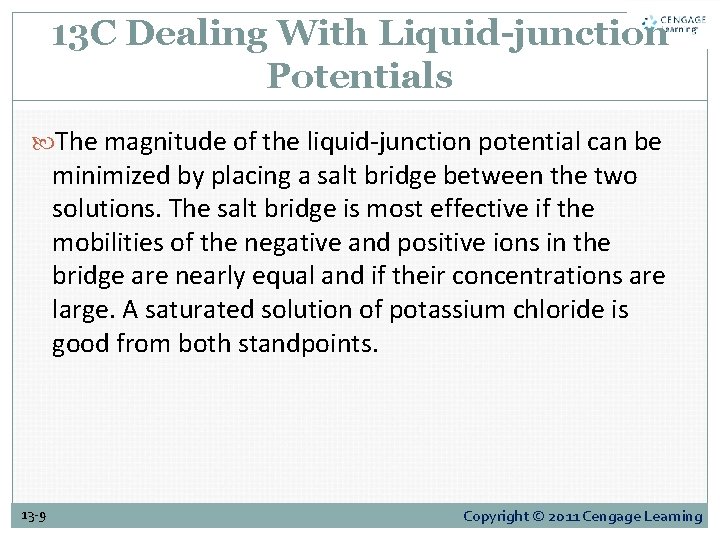
13 C Dealing With Liquid-junction Potentials The magnitude of the liquid-junction potential can be minimized by placing a salt bridge between the two solutions. The salt bridge is most effective if the mobilities of the negative and positive ions in the bridge are nearly equal and if their concentrations are large. A saturated solution of potassium chloride is good from both standpoints. 13 -9 Copyright © 2011 Cengage Learning
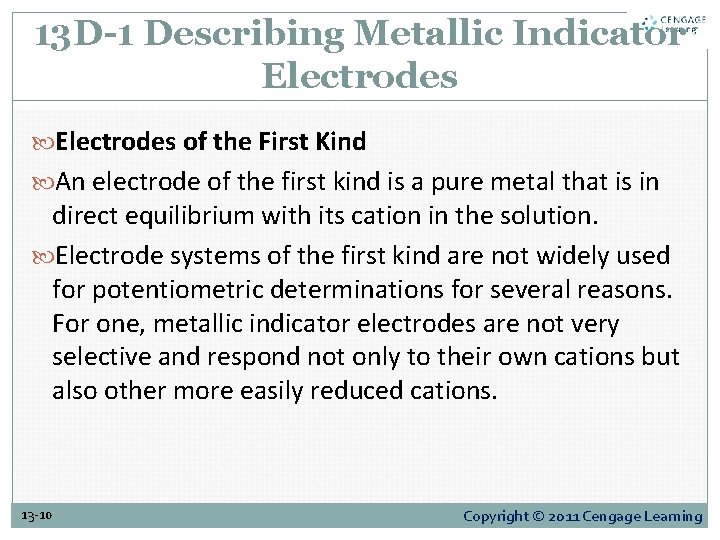
13 D-1 Describing Metallic Indicator Electrodes of the First Kind An electrode of the first kind is a pure metal that is in direct equilibrium with its cation in the solution. Electrode systems of the first kind are not widely used for potentiometric determinations for several reasons. For one, metallic indicator electrodes are not very selective and respond not only to their own cations but also other more easily reduced cations. 13 -10 Copyright © 2011 Cengage Learning
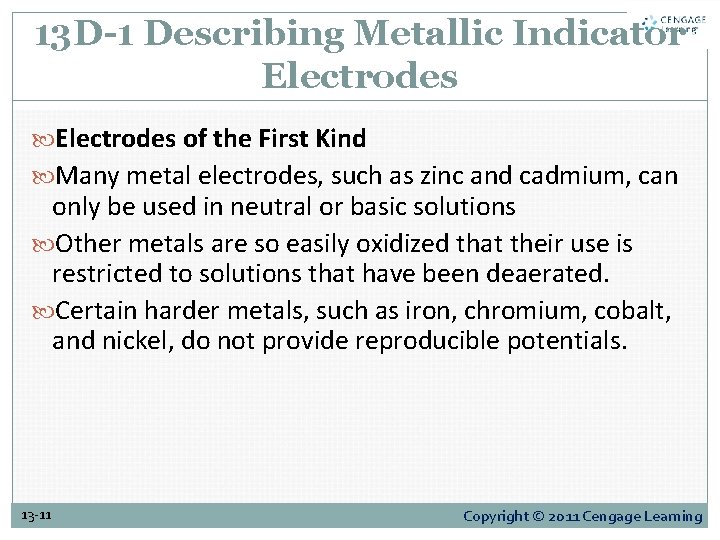
13 D-1 Describing Metallic Indicator Electrodes of the First Kind Many metal electrodes, such as zinc and cadmium, can only be used in neutral or basic solutions Other metals are so easily oxidized that their use is restricted to solutions that have been deaerated. Certain harder metals, such as iron, chromium, cobalt, and nickel, do not provide reproducible potentials. 13 -11 Copyright © 2011 Cengage Learning
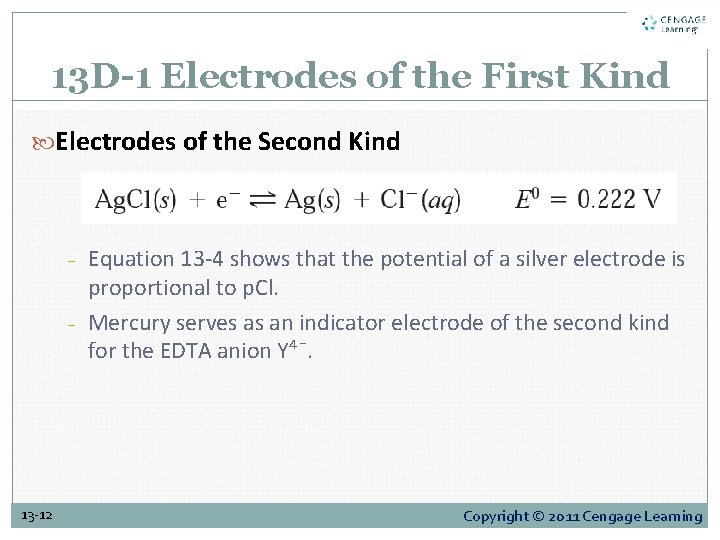
13 D-1 Electrodes of the First Kind Electrodes of the Second Kind − − 13 -12 Equation 13 -4 shows that the potential of a silver electrode is proportional to p. Cl. Mercury serves as an indicator electrode of the second kind for the EDTA anion Y⁴¯. Copyright © 2011 Cengage Learning
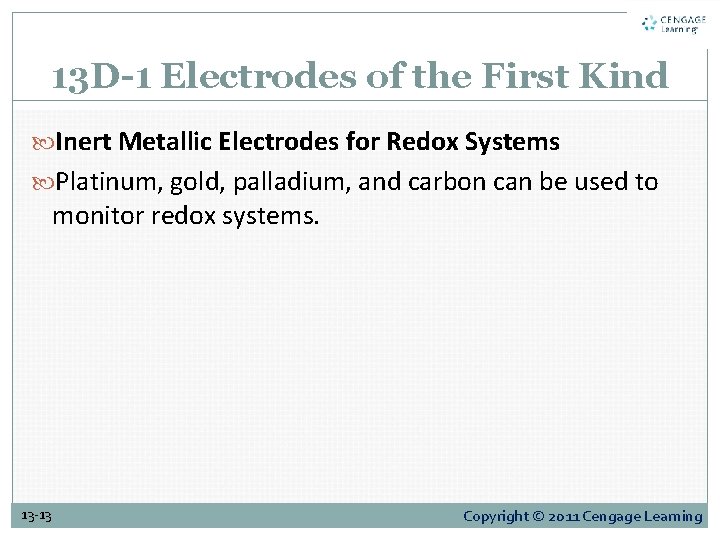
13 D-1 Electrodes of the First Kind Inert Metallic Electrodes for Redox Systems Platinum, gold, palladium, and carbon can be used to monitor redox systems. 13 -13 Copyright © 2011 Cengage Learning
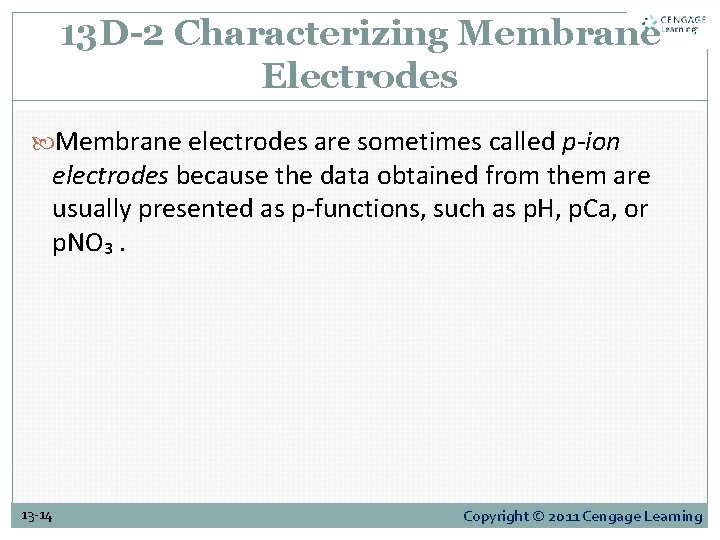
13 D-2 Characterizing Membrane Electrodes Membrane electrodes are sometimes called p-ion electrodes because the data obtained from them are usually presented as p-functions, such as p. H, p. Ca, or p. NO₃. 13 -14 Copyright © 2011 Cengage Learning
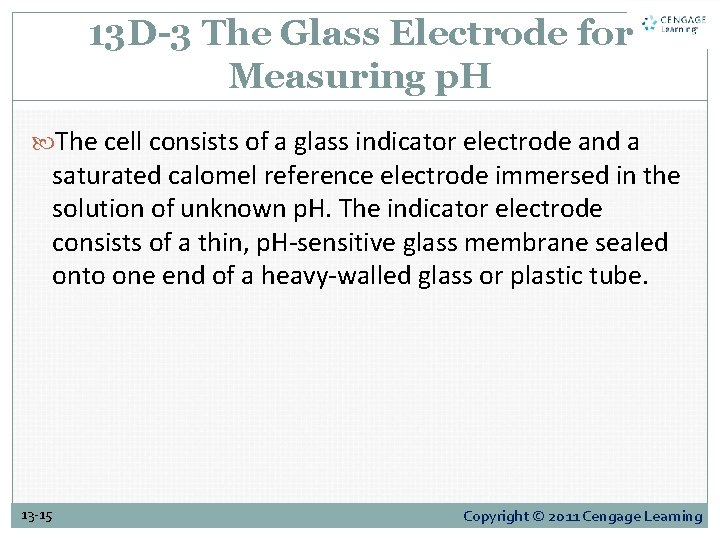
13 D-3 The Glass Electrode for Measuring p. H The cell consists of a glass indicator electrode and a saturated calomel reference electrode immersed in the solution of unknown p. H. The indicator electrode consists of a thin, p. H-sensitive glass membrane sealed onto one end of a heavy-walled glass or plastic tube. 13 -15 Copyright © 2011 Cengage Learning
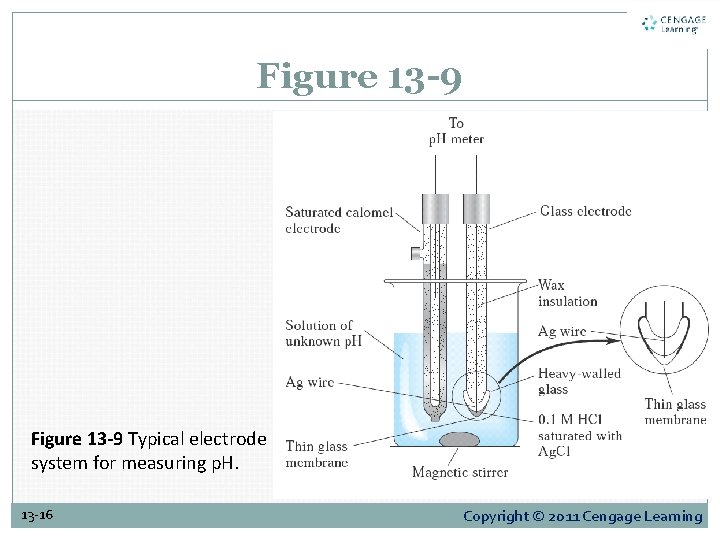
Figure 13 -9 Typical electrode system for measuring p. H. 13 -16 Copyright © 2011 Cengage Learning
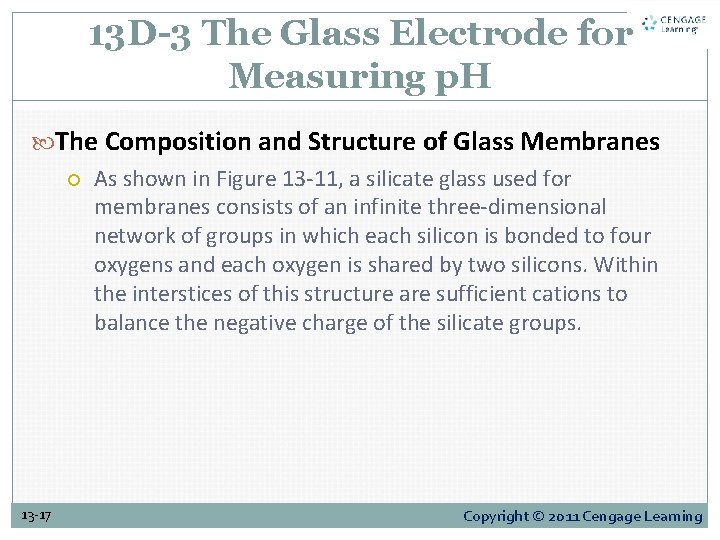
13 D-3 The Glass Electrode for Measuring p. H The Composition and Structure of Glass Membranes As shown in Figure 13 -11, a silicate glass used for membranes consists of an infinite three-dimensional network of groups in which each silicon is bonded to four oxygens and each oxygen is shared by two silicons. Within the interstices of this structure are sufficient cations to balance the negative charge of the silicate groups. 13 -17 Copyright © 2011 Cengage Learning
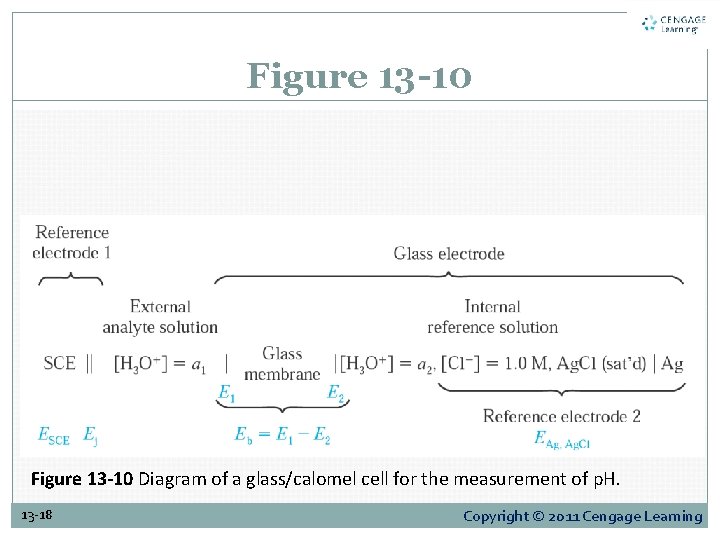
Figure 13 -10 Diagram of a glass/calomel cell for the measurement of p. H. 13 -18 Copyright © 2011 Cengage Learning
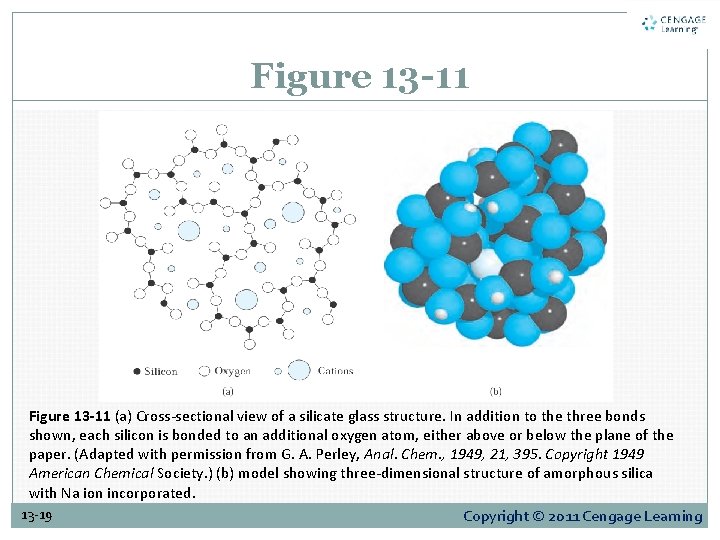
Figure 13 -11 (a) Cross-sectional view of a silicate glass structure. In addition to the three bonds shown, each silicon is bonded to an additional oxygen atom, either above or below the plane of the paper. (Adapted with permission from G. A. Perley, Anal. Chem. , 1949, 21, 395. Copyright 1949 American Chemical Society. ) (b) model showing three-dimensional structure of amorphous silica with Na ion incorporated. 13 -19 Copyright © 2011 Cengage Learning
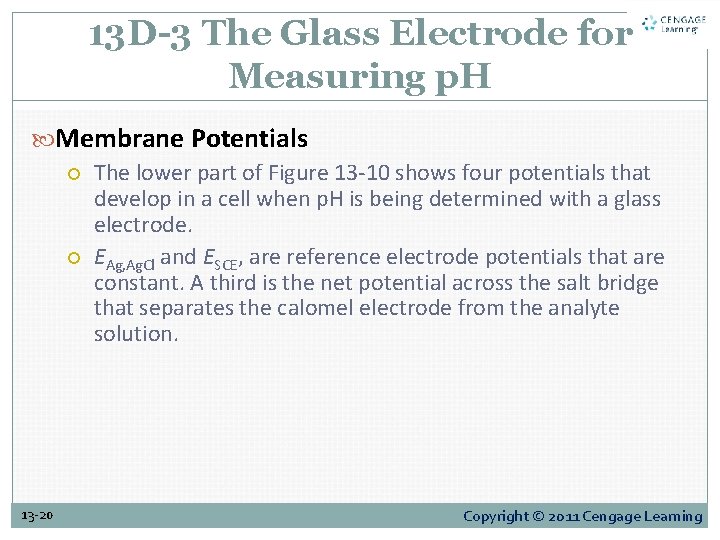
13 D-3 The Glass Electrode for Measuring p. H Membrane Potentials The lower part of Figure 13 -10 shows four potentials that develop in a cell when p. H is being determined with a glass electrode. EAg, Ag. Cl and ESCE, are reference electrode potentials that are constant. A third is the net potential across the salt bridge that separates the calomel electrode from the analyte solution. 13 -20 Copyright © 2011 Cengage Learning
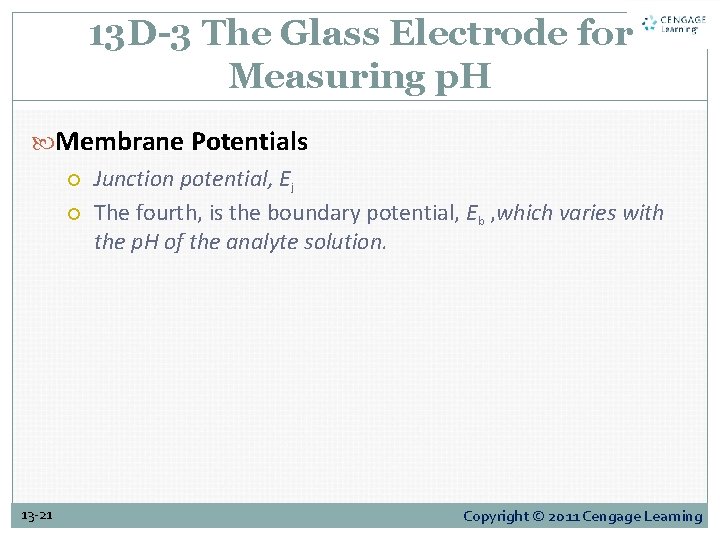
13 D-3 The Glass Electrode for Measuring p. H Membrane Potentials Junction potential, Ej The fourth, is the boundary potential, Eb , which varies with the p. H of the analyte solution. 13 -21 Copyright © 2011 Cengage Learning
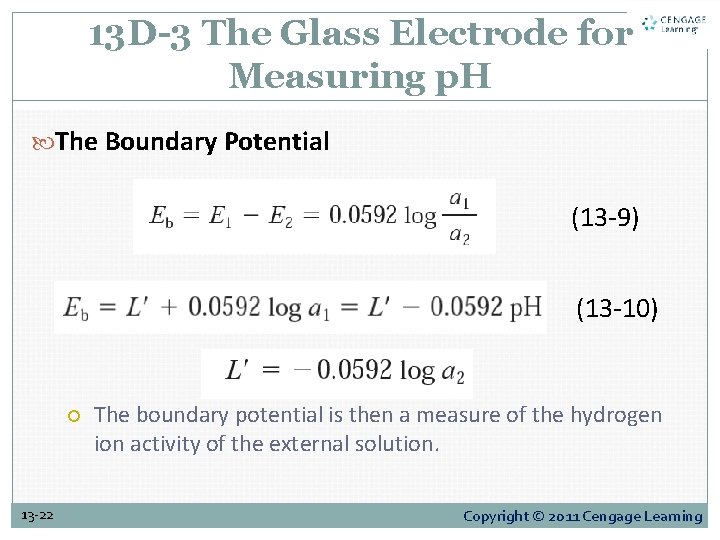
13 D-3 The Glass Electrode for Measuring p. H The Boundary Potential (13 -9) (13 -10) 13 -22 The boundary potential is then a measure of the hydrogen ion activity of the external solution. Copyright © 2011 Cengage Learning
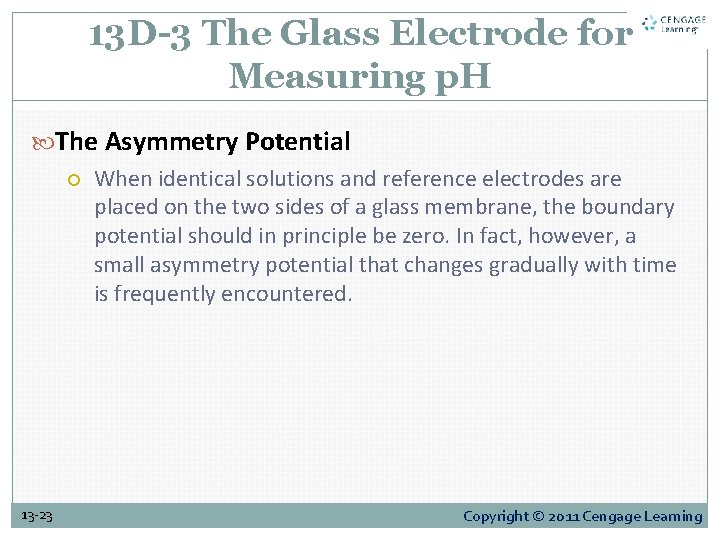
13 D-3 The Glass Electrode for Measuring p. H The Asymmetry Potential When identical solutions and reference electrodes are placed on the two sides of a glass membrane, the boundary potential should in principle be zero. In fact, however, a small asymmetry potential that changes gradually with time is frequently encountered. 13 -23 Copyright © 2011 Cengage Learning
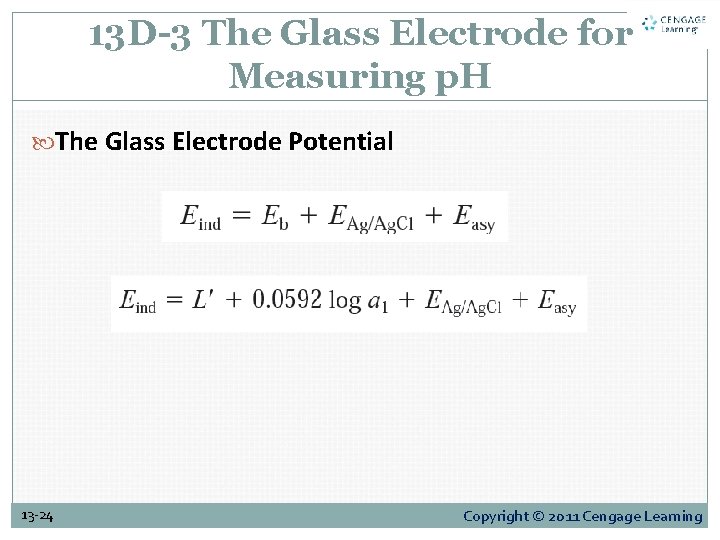
13 D-3 The Glass Electrode for Measuring p. H The Glass Electrode Potential 13 -24 Copyright © 2011 Cengage Learning
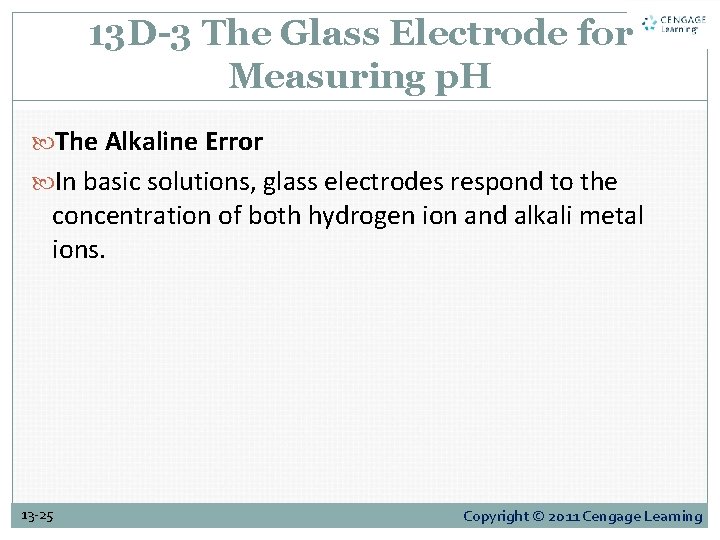
13 D-3 The Glass Electrode for Measuring p. H The Alkaline Error In basic solutions, glass electrodes respond to the concentration of both hydrogen ion and alkali metal ions. 13 -25 Copyright © 2011 Cengage Learning
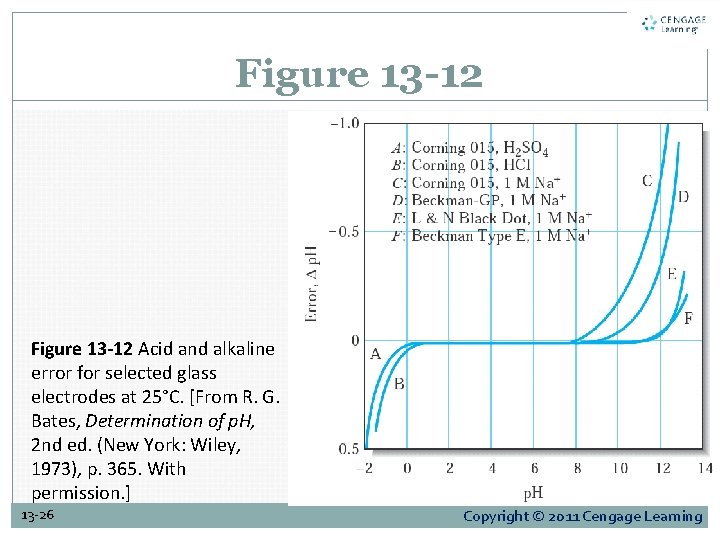
Figure 13 -12 Acid and alkaline error for selected glass electrodes at 25°C. [From R. G. Bates, Determination of p. H, 2 nd ed. (New York: Wiley, 1973), p. 365. With permission. ] 13 -26 Copyright © 2011 Cengage Learning
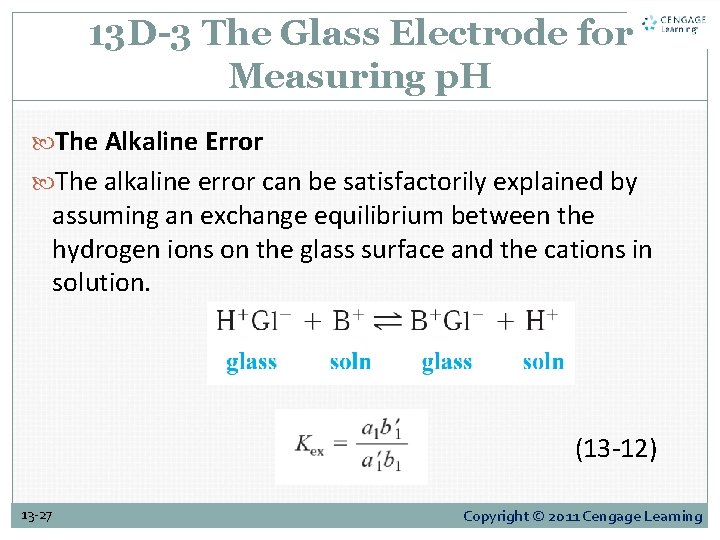
13 D-3 The Glass Electrode for Measuring p. H The Alkaline Error The alkaline error can be satisfactorily explained by assuming an exchange equilibrium between the hydrogen ions on the glass surface and the cations in solution. (13 -12) 13 -27 Copyright © 2011 Cengage Learning
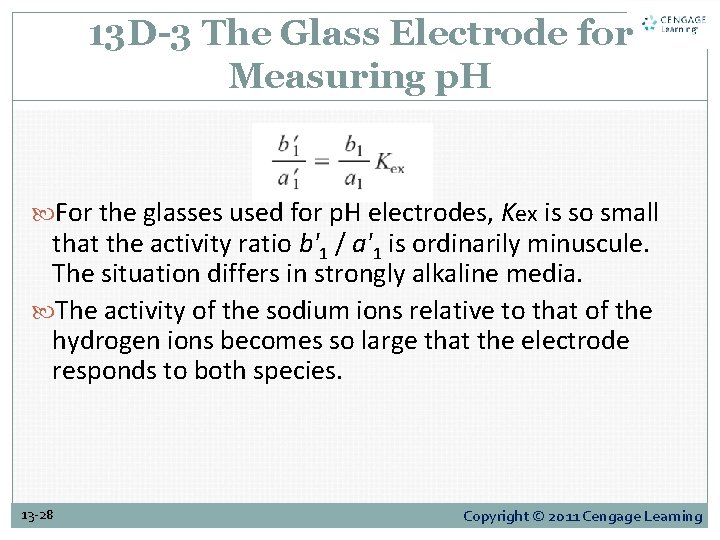
13 D-3 The Glass Electrode for Measuring p. H For the glasses used for p. H electrodes, Kex is so small that the activity ratio b'1 / a'1 is ordinarily minuscule. The situation differs in strongly alkaline media. The activity of the sodium ions relative to that of the hydrogen ions becomes so large that the electrode responds to both species. 13 -28 Copyright © 2011 Cengage Learning
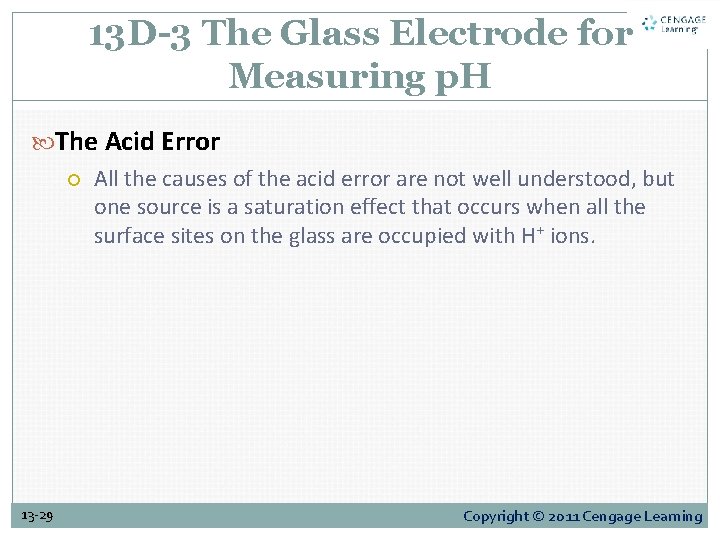
13 D-3 The Glass Electrode for Measuring p. H The Acid Error All the causes of the acid error are not well understood, but one source is a saturation effect that occurs when all the surface sites on the glass are occupied with H⁺ ions. 13 -29 Copyright © 2011 Cengage Learning
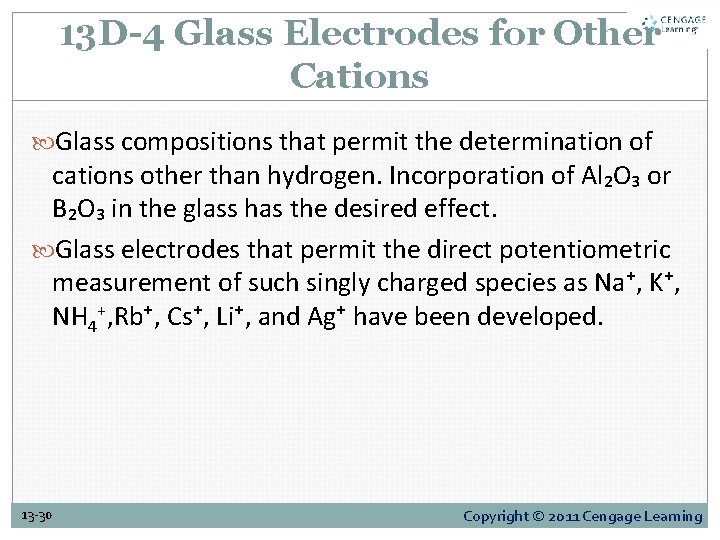
13 D-4 Glass Electrodes for Other Cations Glass compositions that permit the determination of cations other than hydrogen. Incorporation of Al₂O₃ or B₂O₃ in the glass has the desired effect. Glass electrodes that permit the direct potentiometric measurement of such singly charged species as Na⁺, K⁺, NH 4+, Rb⁺, Cs⁺, Li⁺, and Ag⁺ have been developed. 13 -30 Copyright © 2011 Cengage Learning
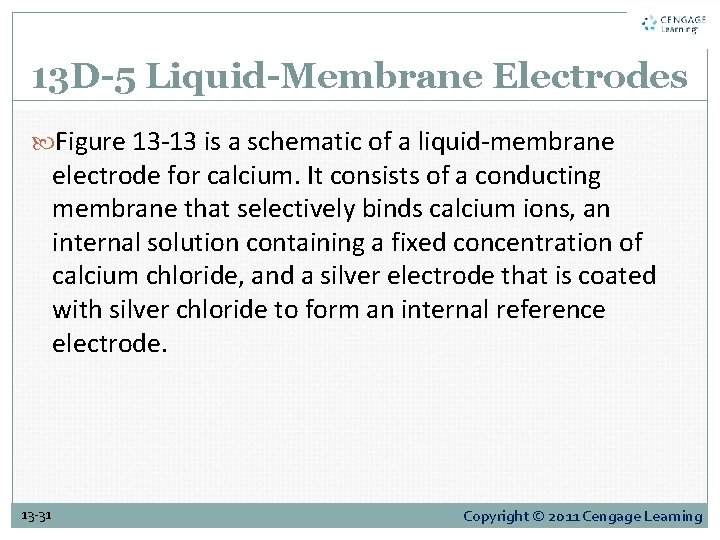
13 D-5 Liquid-Membrane Electrodes Figure 13 -13 is a schematic of a liquid-membrane electrode for calcium. It consists of a conducting membrane that selectively binds calcium ions, an internal solution containing a fixed concentration of calcium chloride, and a silver electrode that is coated with silver chloride to form an internal reference electrode. 13 -31 Copyright © 2011 Cengage Learning
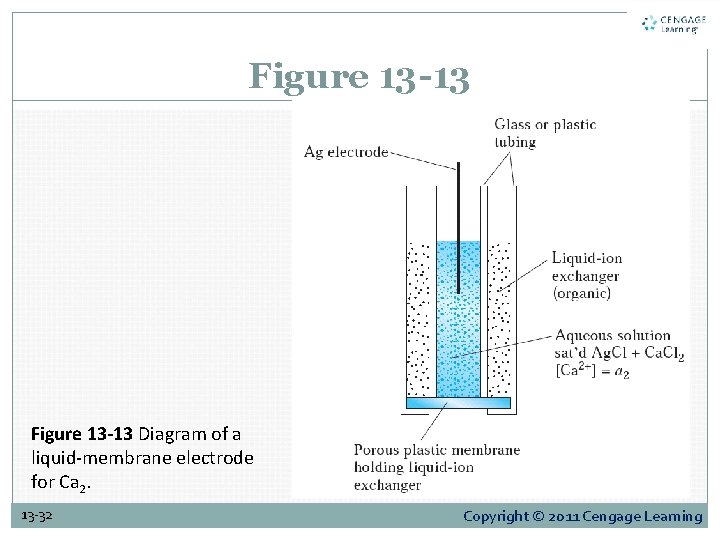
Figure 13 -13 Diagram of a liquid-membrane electrode for Ca 2. 13 -32 Copyright © 2011 Cengage Learning
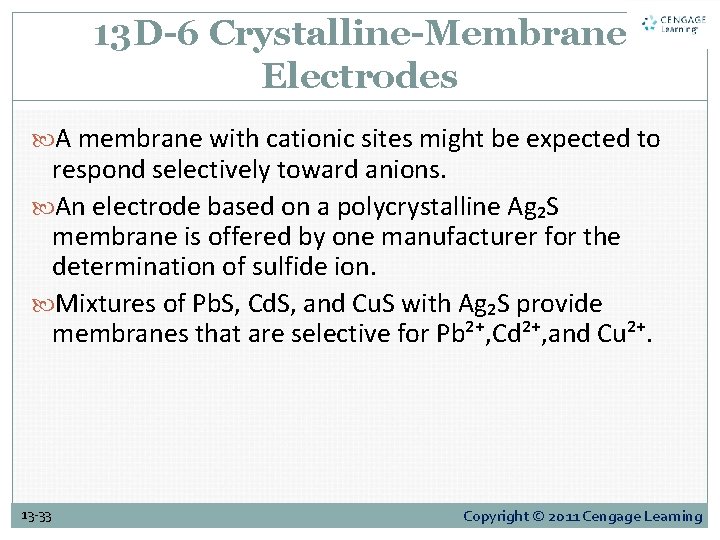
13 D-6 Crystalline-Membrane Electrodes A membrane with cationic sites might be expected to respond selectively toward anions. An electrode based on a polycrystalline Ag₂S membrane is offered by one manufacturer for the determination of sulfide ion. Mixtures of Pb. S, Cd. S, and Cu. S with Ag₂S provide membranes that are selective for Pb²⁺, Cd²⁺, and Cu²⁺. 13 -33 Copyright © 2011 Cengage Learning
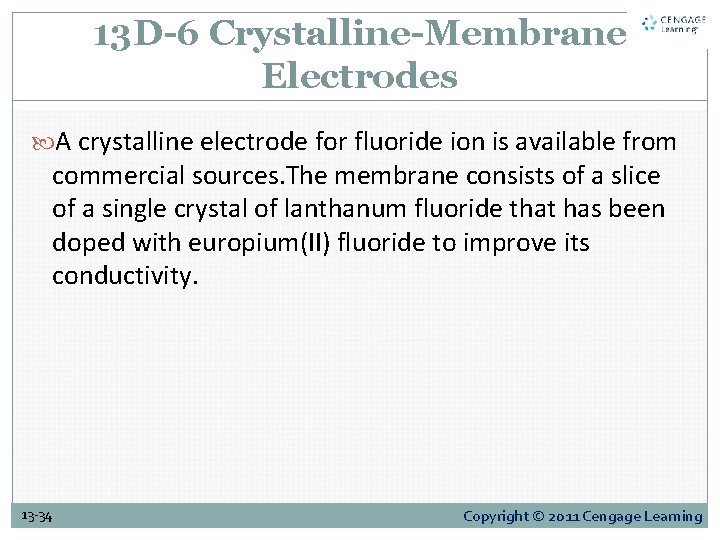
13 D-6 Crystalline-Membrane Electrodes A crystalline electrode for fluoride ion is available from commercial sources. The membrane consists of a slice of a single crystal of lanthanum fluoride that has been doped with europium(II) fluoride to improve its conductivity. 13 -34 Copyright © 2011 Cengage Learning
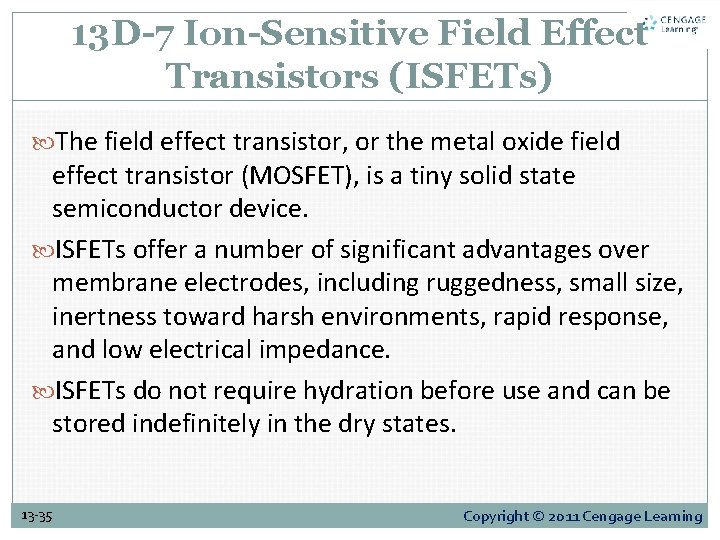
13 D-7 Ion-Sensitive Field Effect Transistors (ISFETs) The field effect transistor, or the metal oxide field effect transistor (MOSFET), is a tiny solid state semiconductor device. ISFETs offer a number of significant advantages over membrane electrodes, including ruggedness, small size, inertness toward harsh environments, rapid response, and low electrical impedance. ISFETs do not require hydration before use and can be stored indefinitely in the dry states. 13 -35 Copyright © 2011 Cengage Learning
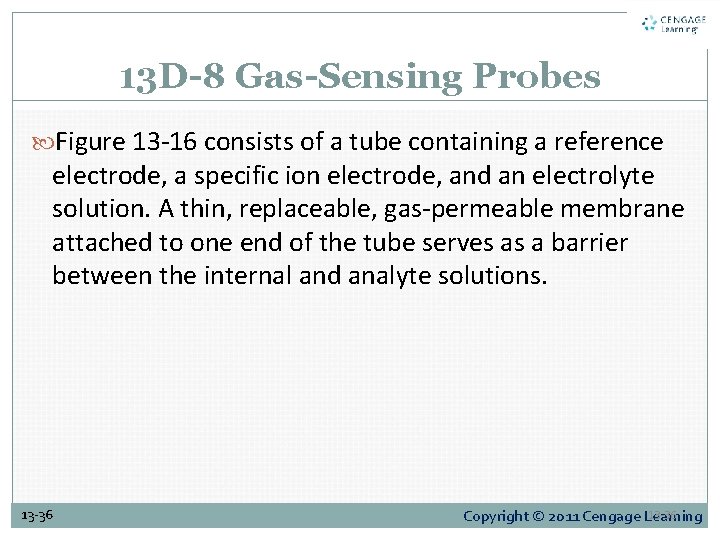
13 D-8 Gas-Sensing Probes Figure 13 -16 consists of a tube containing a reference electrode, a specific ion electrode, and an electrolyte solution. A thin, replaceable, gas-permeable membrane attached to one end of the tube serves as a barrier between the internal and analyte solutions. 13 -36 Copyright © 2011 Cengage Learning
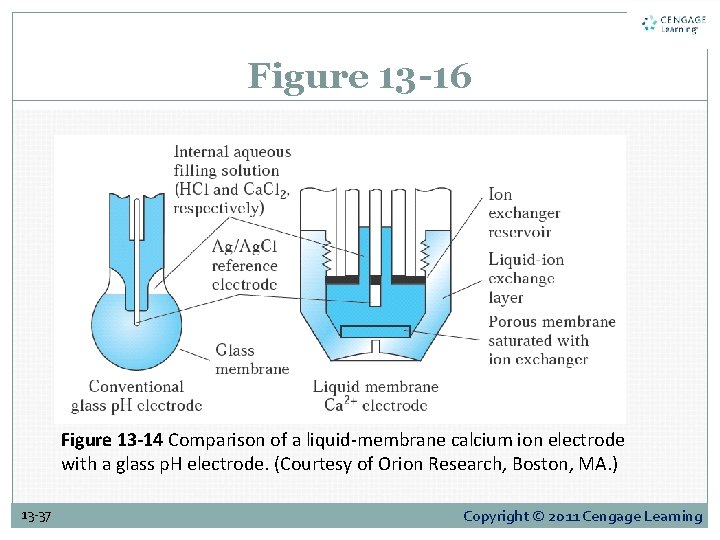
Figure 13 -16 Figure 13 -14 Comparison of a liquid-membrane calcium ion electrode with a glass p. H electrode. (Courtesy of Orion Research, Boston, MA. ) 13 -37 Copyright © 2011 Cengage Learning
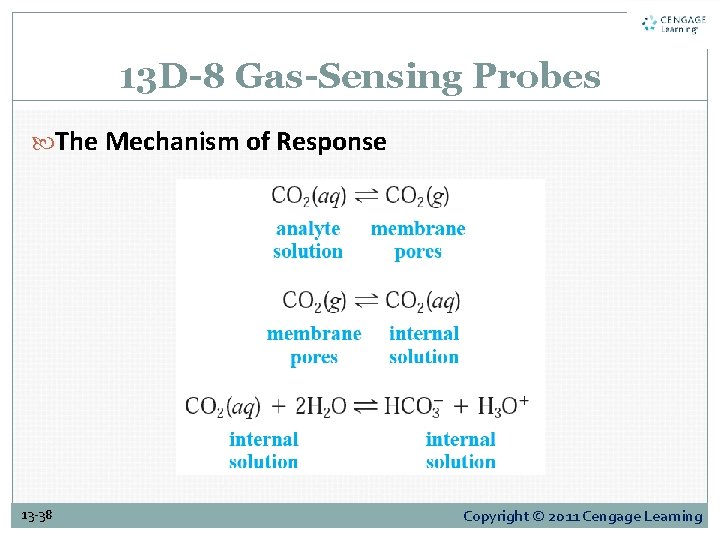
13 D-8 Gas-Sensing Probes The Mechanism of Response 13 -38 Copyright © 2011 Cengage Learning
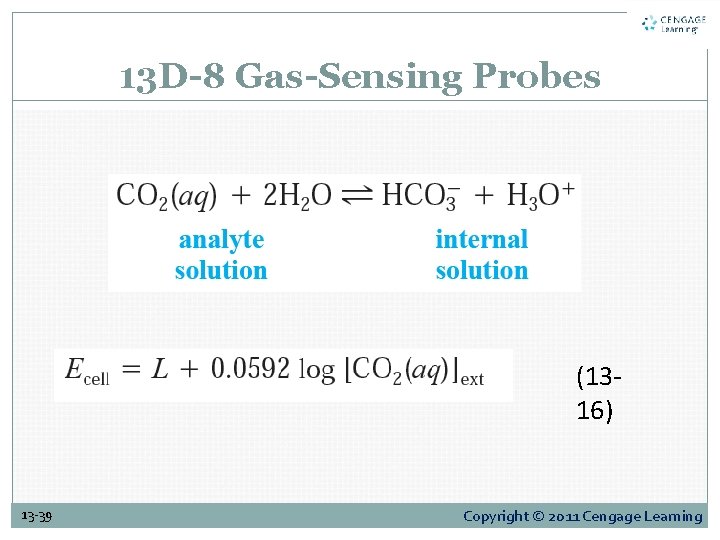
13 D-8 Gas-Sensing Probes (1316) 13 -39 Copyright © 2011 Cengage Learning
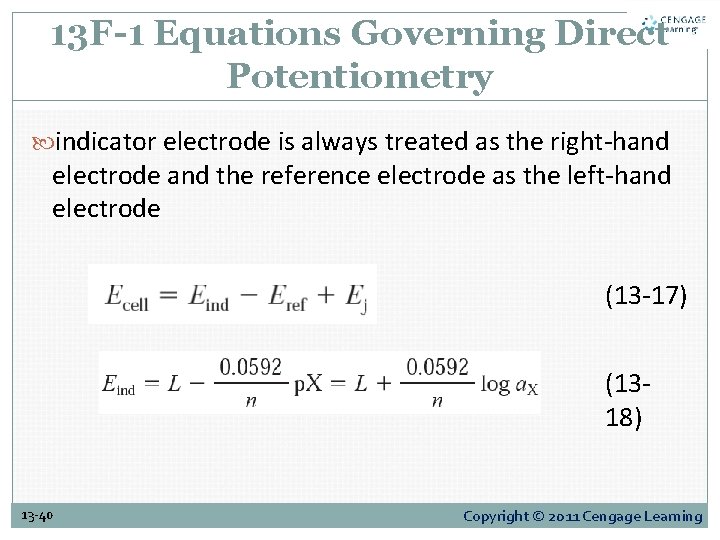
13 F-1 Equations Governing Direct Potentiometry indicator electrode is always treated as the right-hand electrode and the reference electrode as the left-hand electrode (13 -17) (1318) 13 -40 Copyright © 2011 Cengage Learning
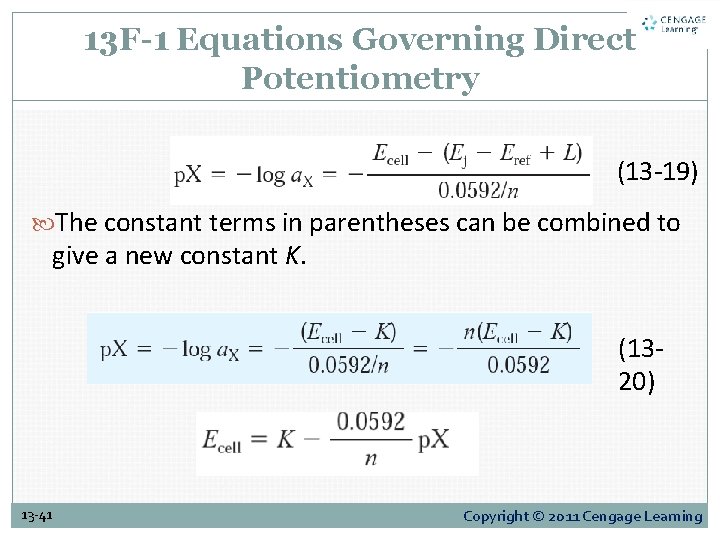
13 F-1 Equations Governing Direct Potentiometry (13 -19) The constant terms in parentheses can be combined to give a new constant K. (1320) 13 -41 Copyright © 2011 Cengage Learning
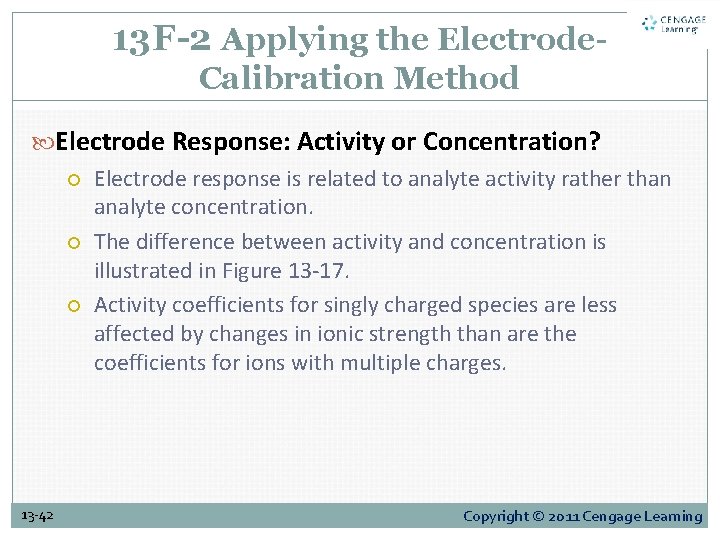
13 F-2 Applying the Electrode. Calibration Method Electrode Response: Activity or Concentration? Electrode response is related to analyte activity rather than analyte concentration. The difference between activity and concentration is illustrated in Figure 13 -17. Activity coefficients for singly charged species are less affected by changes in ionic strength than are the coefficients for ions with multiple charges. 13 -42 Copyright © 2011 Cengage Learning
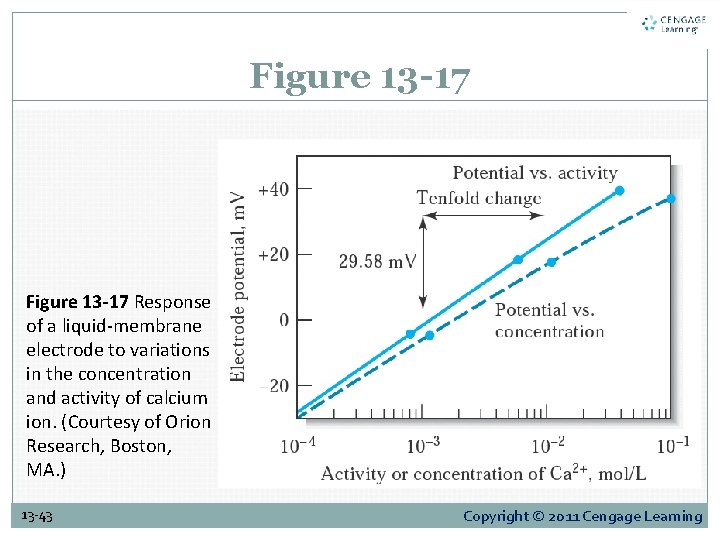
Figure 13 -17 Response of a liquid-membrane electrode to variations in the concentration and activity of calcium ion. (Courtesy of Orion Research, Boston, MA. ) 13 -43 Copyright © 2011 Cengage Learning
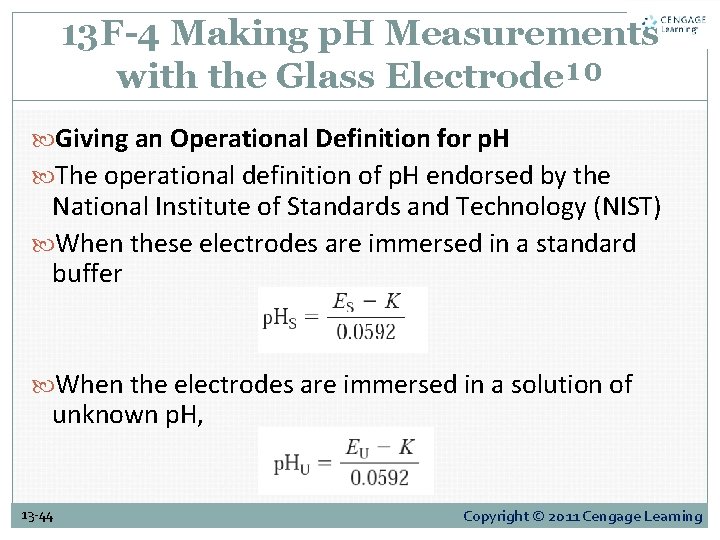
13 F-4 Making p. H Measurements with the Glass Electrode¹⁰ Giving an Operational Definition for p. H The operational definition of p. H endorsed by the National Institute of Standards and Technology (NIST) When these electrodes are immersed in a standard buffer When the electrodes are immersed in a solution of unknown p. H, 13 -44 Copyright © 2011 Cengage Learning
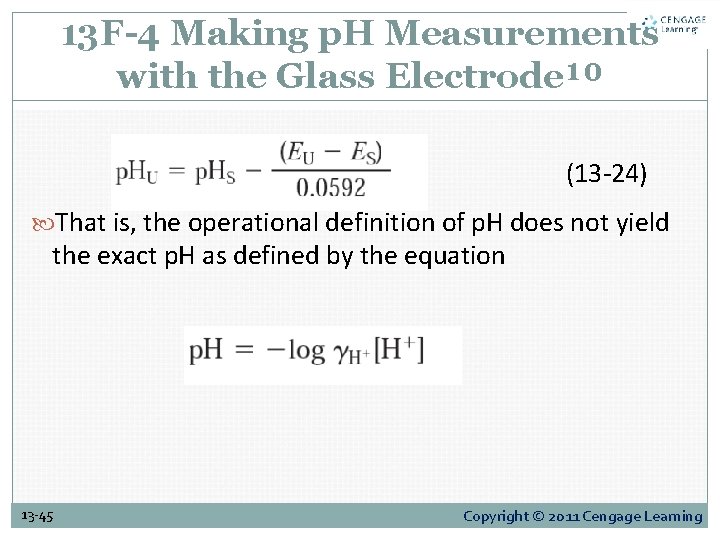
13 F-4 Making p. H Measurements with the Glass Electrode¹⁰ (13 -24) That is, the operational definition of p. H does not yield the exact p. H as defined by the equation 13 -45 Copyright © 2011 Cengage Learning
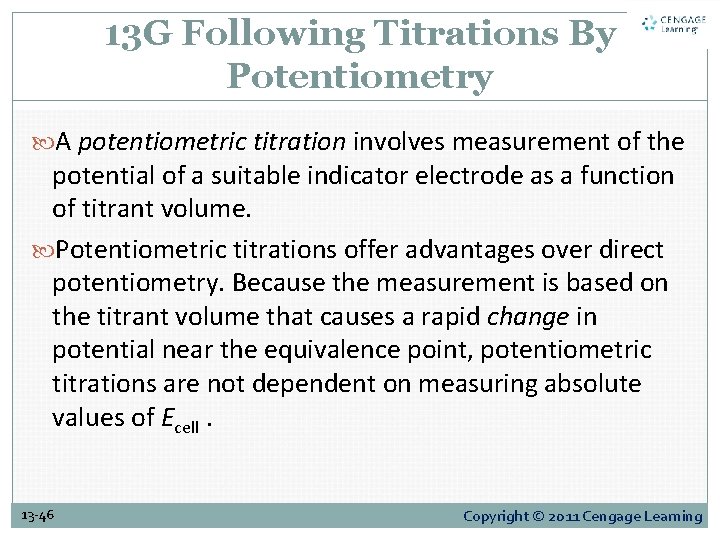
13 G Following Titrations By Potentiometry A potentiometric titration involves measurement of the potential of a suitable indicator electrode as a function of titrant volume. Potentiometric titrations offer advantages over direct potentiometry. Because the measurement is based on the titrant volume that causes a rapid change in potential near the equivalence point, potentiometric titrations are not dependent on measuring absolute values of Ecell. 13 -46 Copyright © 2011 Cengage Learning
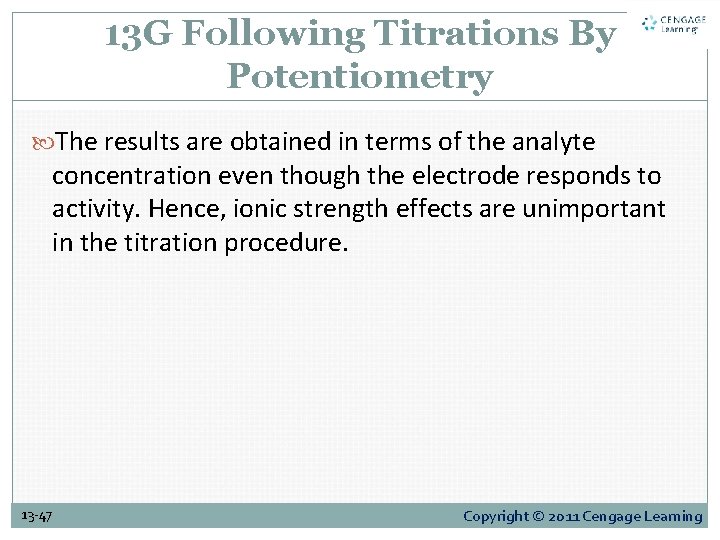
13 G Following Titrations By Potentiometry The results are obtained in terms of the analyte concentration even though the electrode responds to activity. Hence, ionic strength effects are unimportant in the titration procedure. 13 -47 Copyright © 2011 Cengage Learning
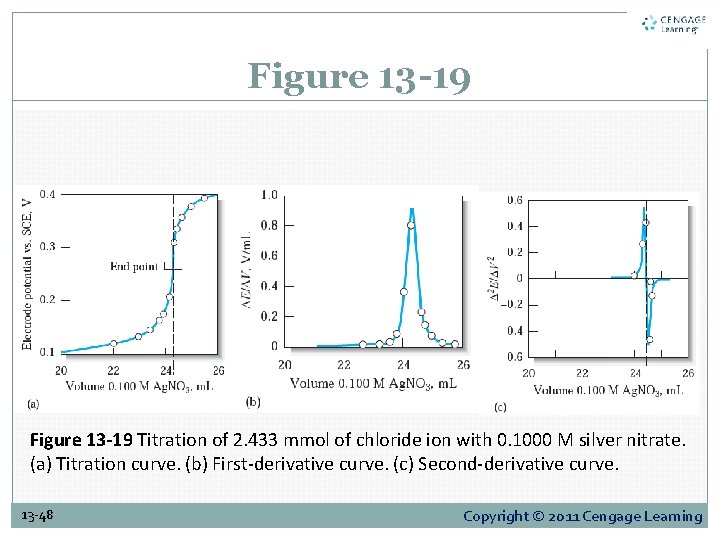
Figure 13 -19 Titration of 2. 433 mmol of chloride ion with 0. 1000 M silver nitrate. (a) Titration curve. (b) First-derivative curve. (c) Second-derivative curve. 13 -48 Copyright © 2011 Cengage Learning
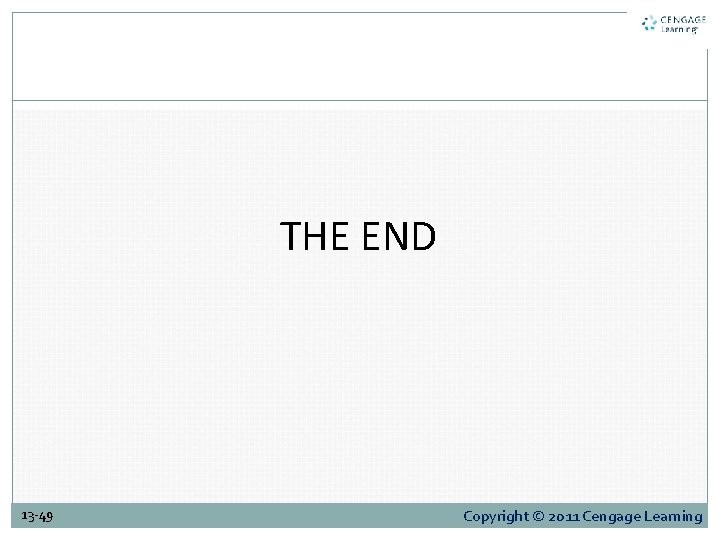
THE END 13 -49 Copyright © 2011 Cengage Learning
Measuring apparatus in analytical chemistry
Potentiometry introduction
Introduction to analytical chemistry
Coprecipitation errors
Organic chemistry chapter 1 problem 59pp
Analytical measuring range
Which cell is used in potentiometry
Potential of the glass electrode is given by
Amperometry vs potentiometry
Difference between conductometry and potentiometry
Direct potentiometry principle
Finomation
Dropping mercury electrode diagram
Potentiometry
Potentiometry
Potentiometry
Difference between voltammetry and potentiometry
Analytical chemistry definition
Chemical error
Jelaskan kegunaan statistika dalam analisis kimia
Normal error curve in analytical chemistry
Redox reaction in alkaline medium
Correlation coefficient in analytical chemistry
Example of analytical chemistry
Q test in analytical chemistry
Annual review of analytical chemistry
Correlation coefficient in analytical chemistry
Analytical chemistry
Excellence in analytical chemistry
Chapter 1 introduction to chemistry
Introduction to chemistry chapter 1
Partition chromatography
Immiscible
Purity
What is an embedded quote
Functional groups ib chemistry
Inorganic chemistry vs organic chemistry
Unit 14.1 measuring and recording vital signs
Tools and measuring instruments chapter 2
Describing motion section 1 answer key
Chapter 29 measuring vital signs
Copyright
Chapter 16.1 measuring and recording vital signs answer key
16:3 measuring and recording pulse
Why are vital signs recorded on a graphic record
Chapter 21:1 measuring/recording height and weight
Describing motion chapter 1 lesson 1 answer key
Chapter 16:1 measuring and recording vital signs
Measuring and constructing segments
Respiratory number 18