Potentiometry and IonSelective Electrodes Lecture 2 1 Emergence
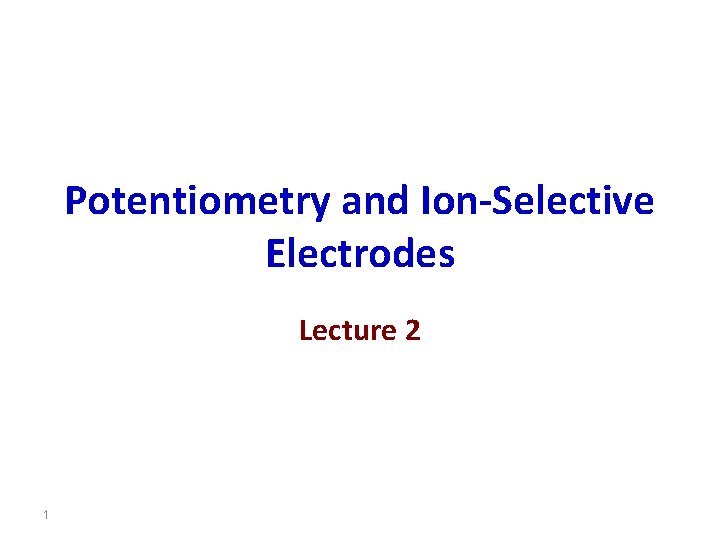
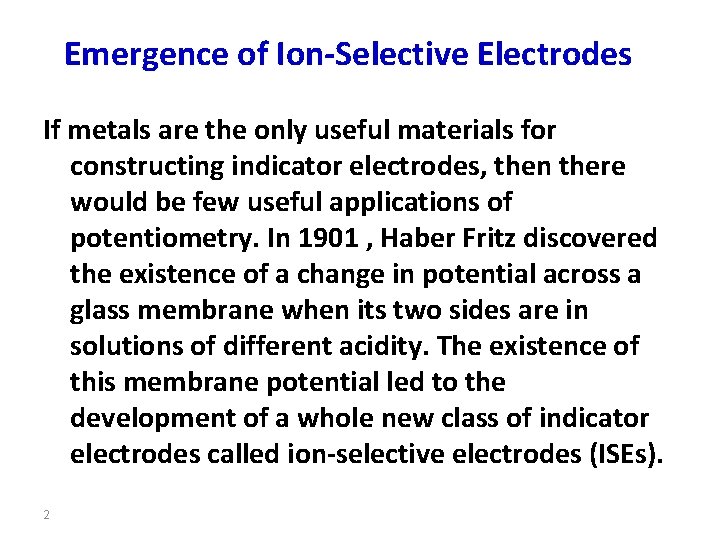
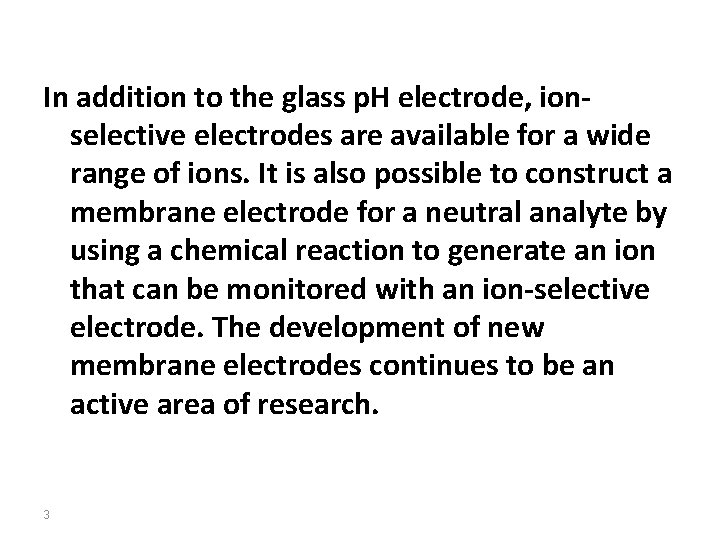
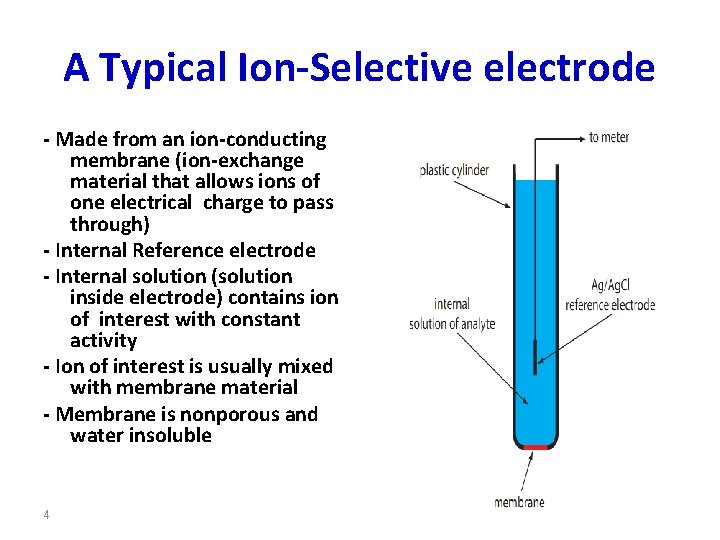
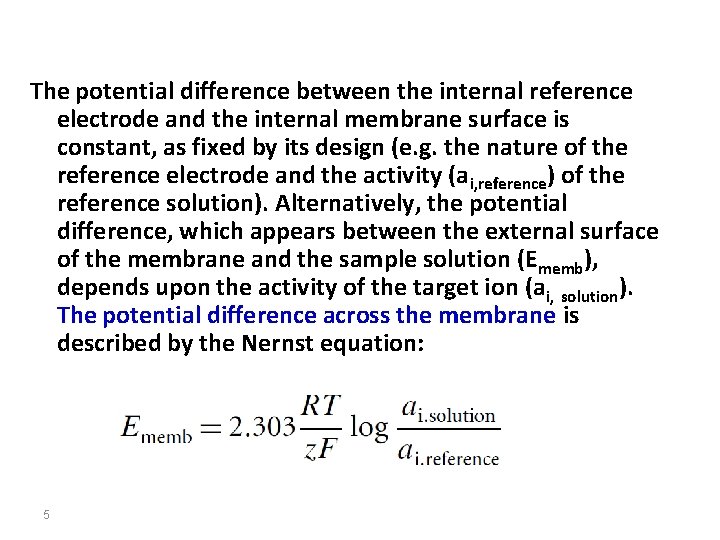
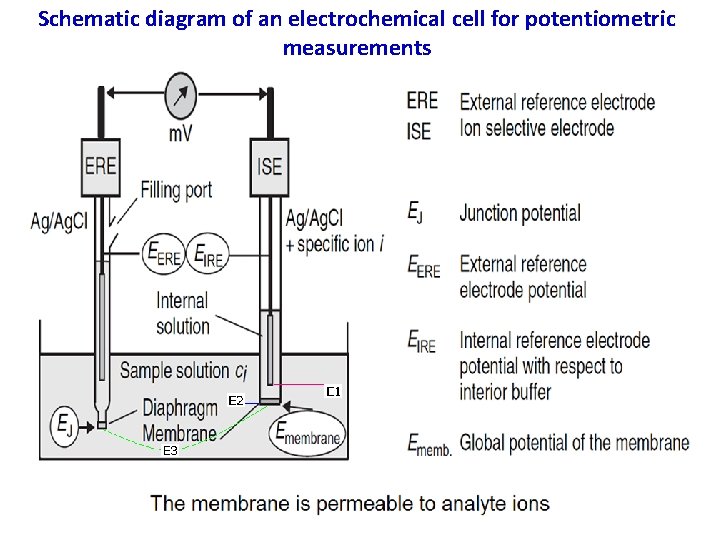
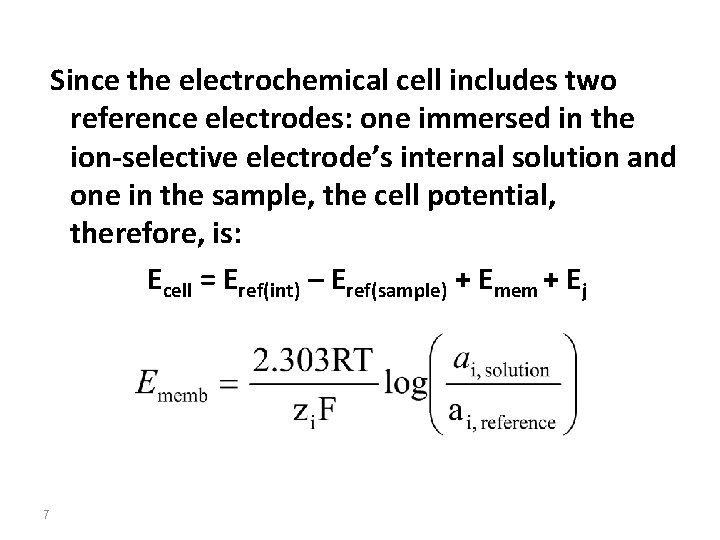
![ION-SELECTIVE ELECTRODES (ISE) - [Analyte ion, i] inside the electrode ≠ [analyte ion, i] ION-SELECTIVE ELECTRODES (ISE) - [Analyte ion, i] inside the electrode ≠ [analyte ion, i]](https://slidetodoc.com/presentation_image/3ae7c1ec86985151055d455023484592/image-8.jpg)
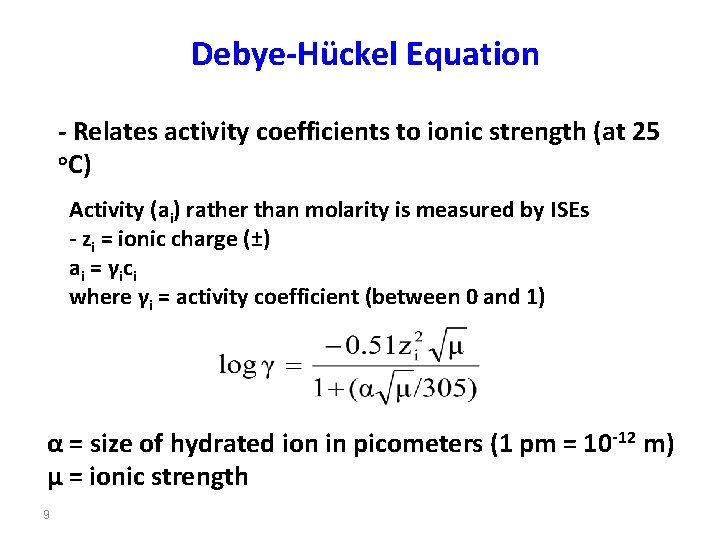
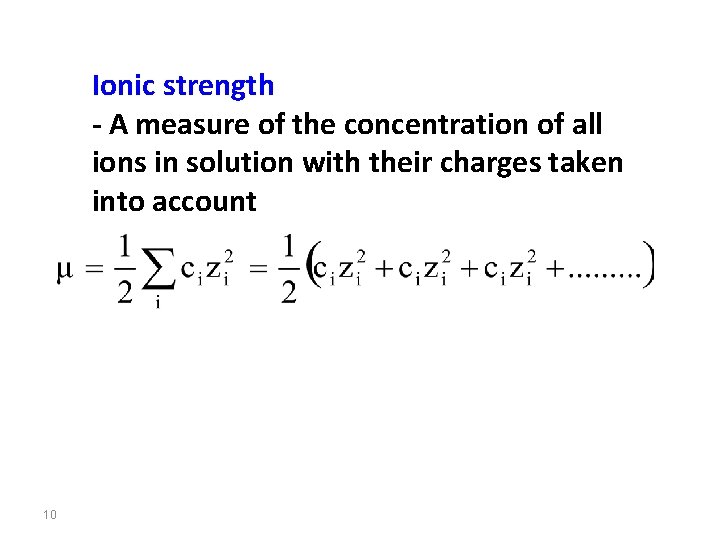
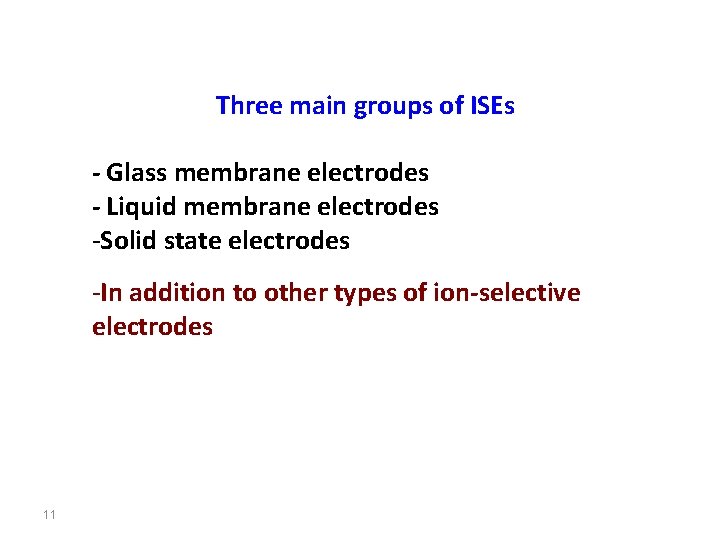
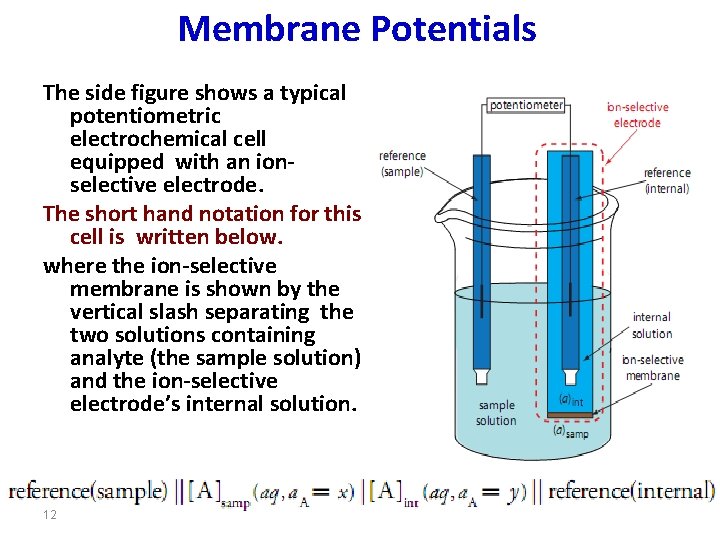
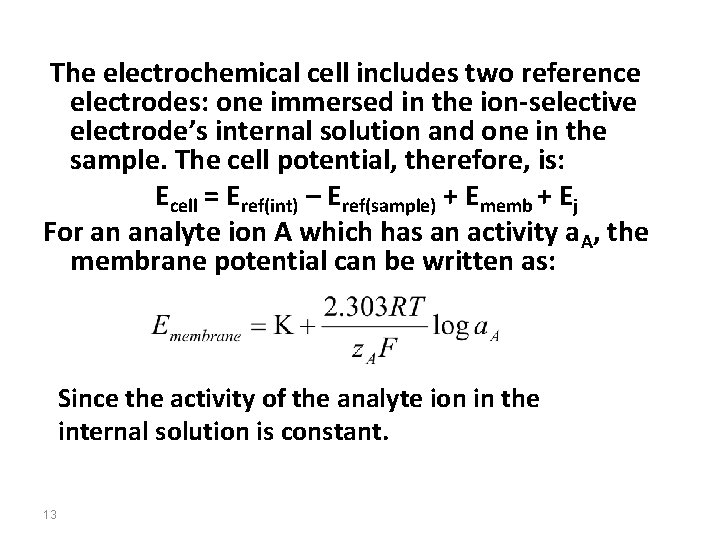
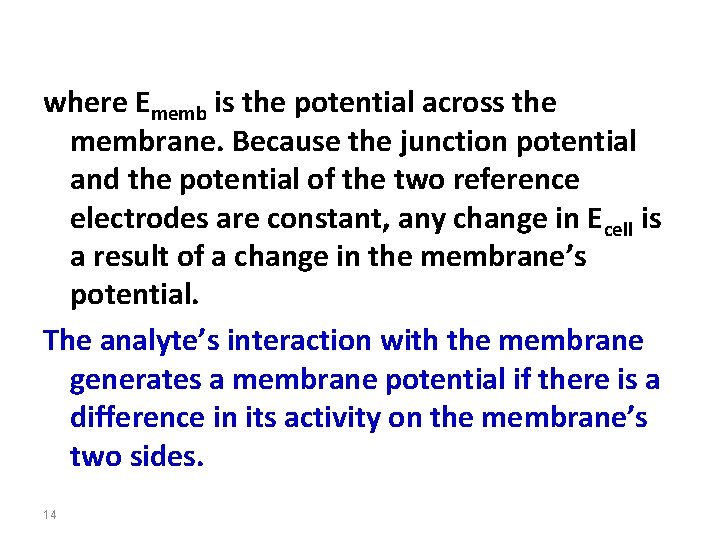
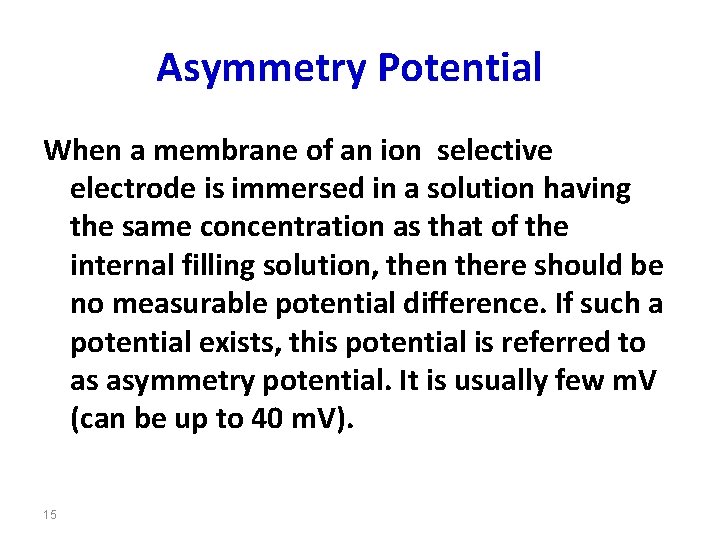
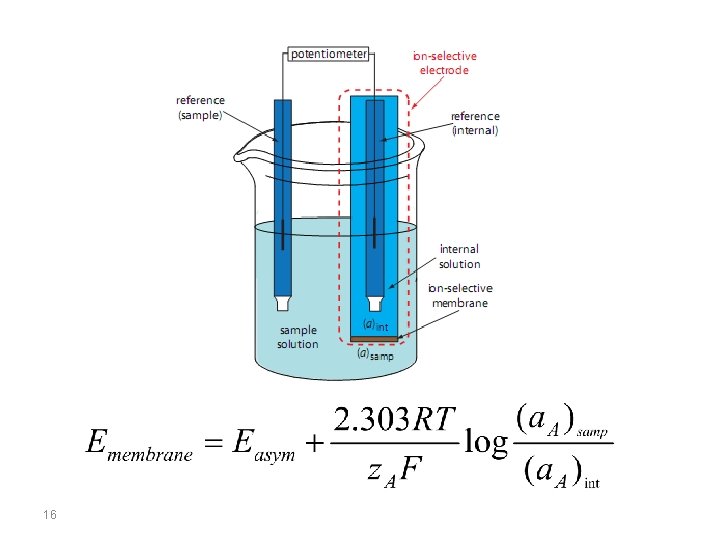
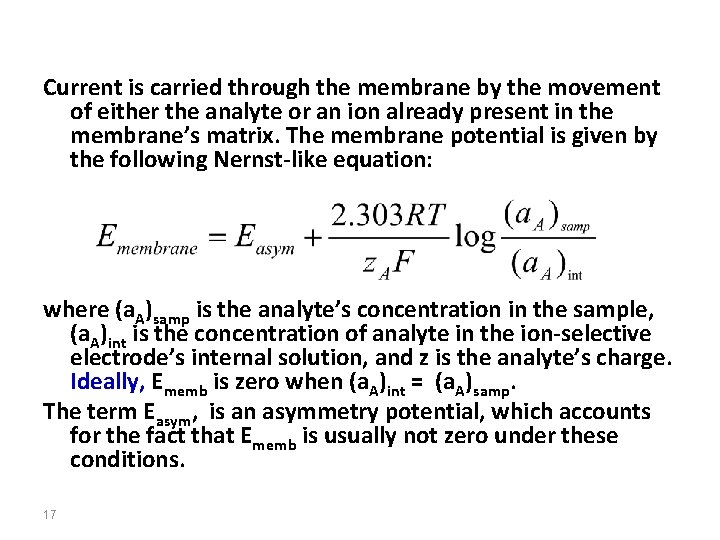
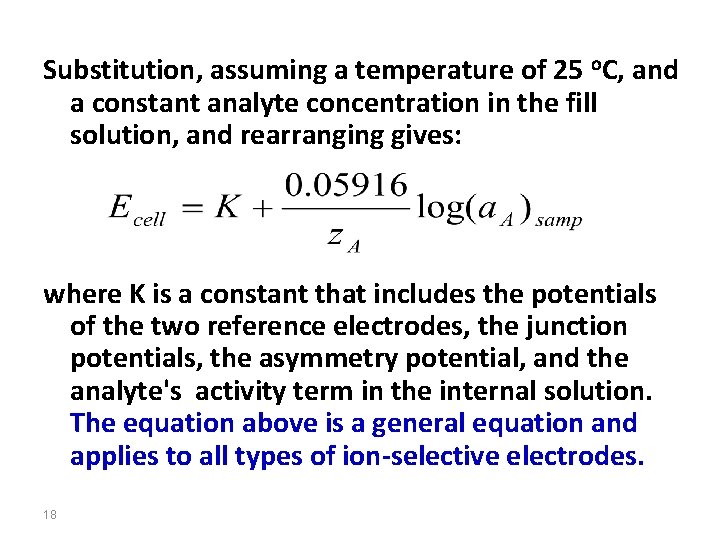
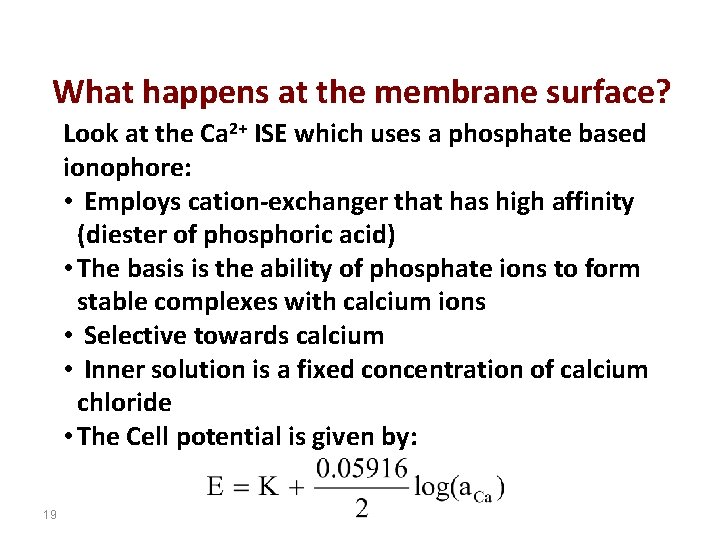
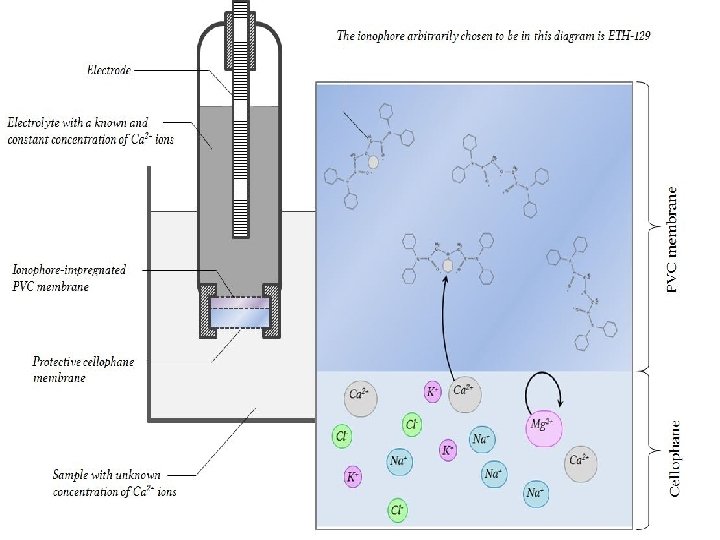
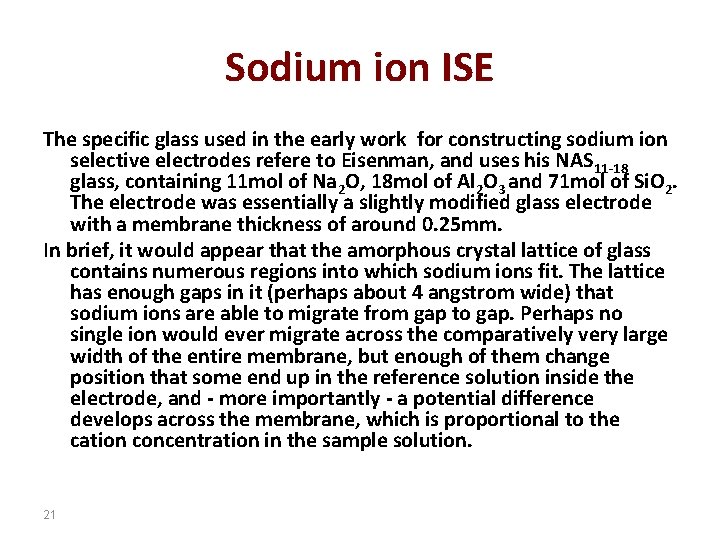
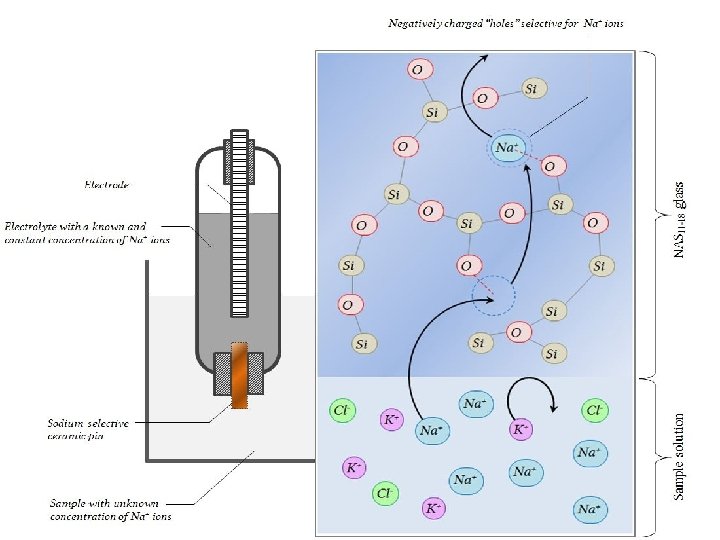
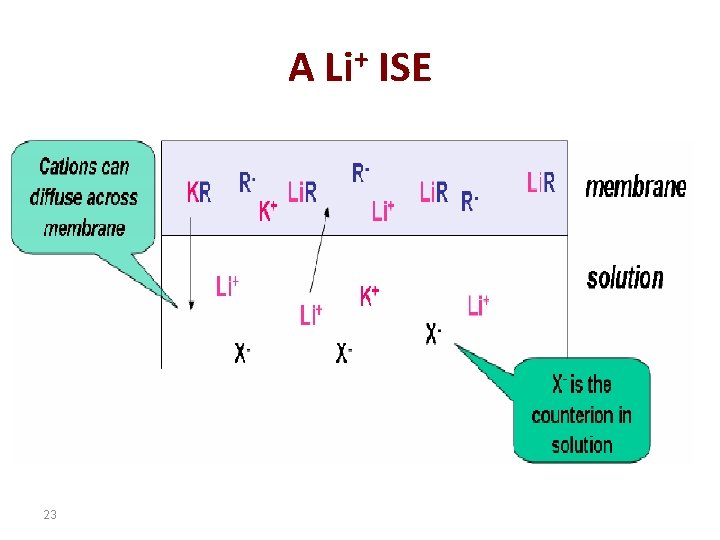
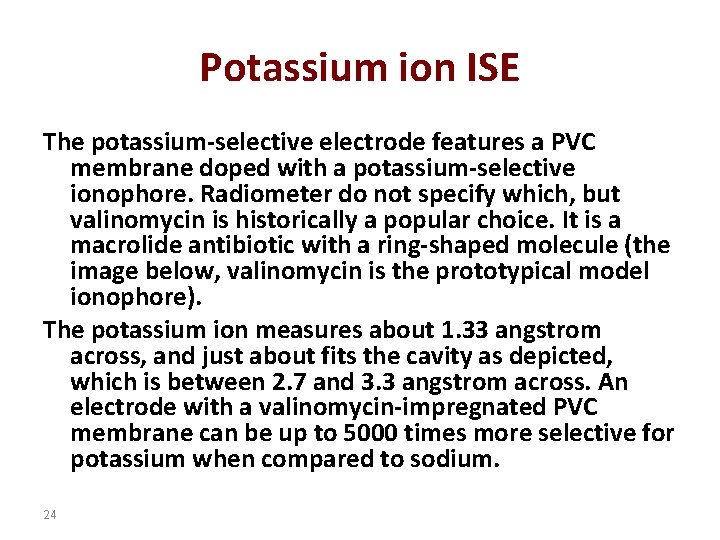
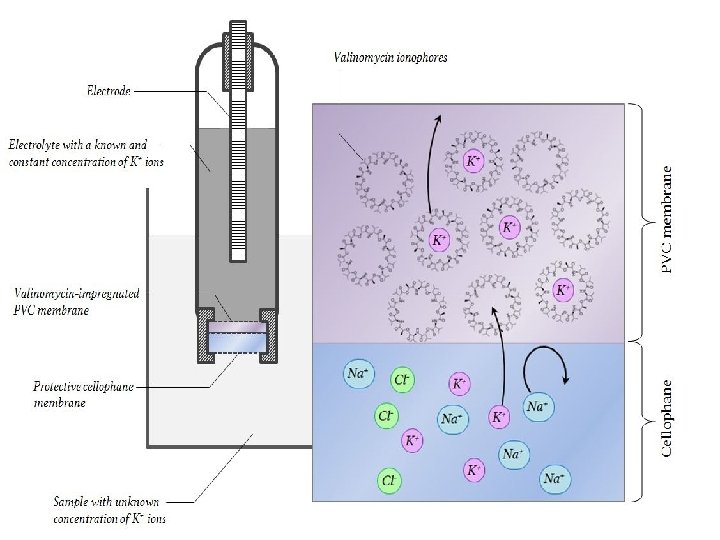
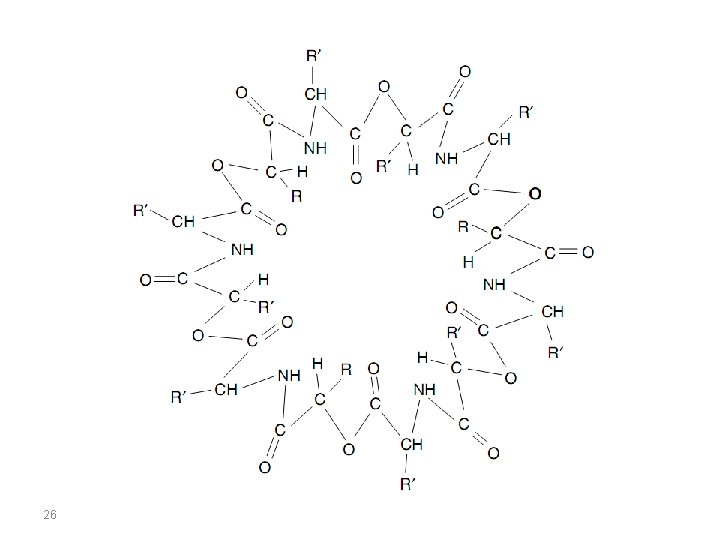
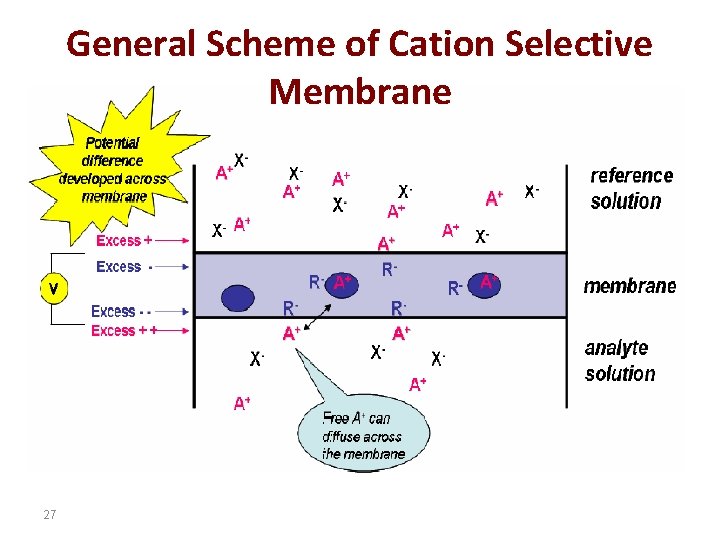
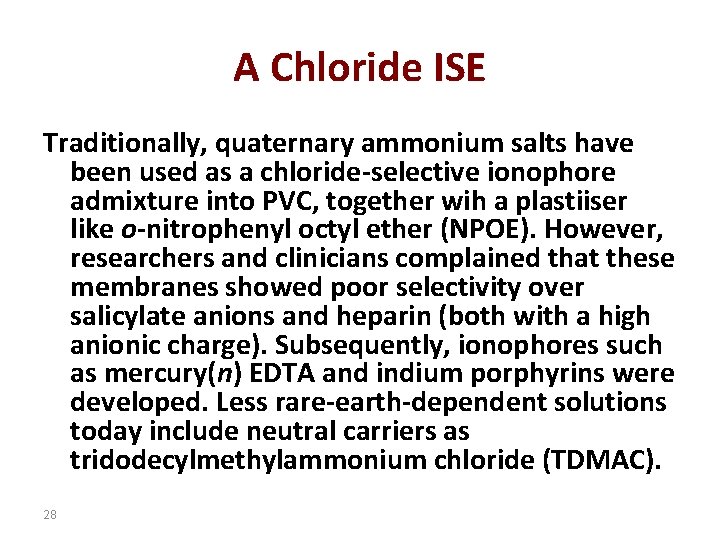
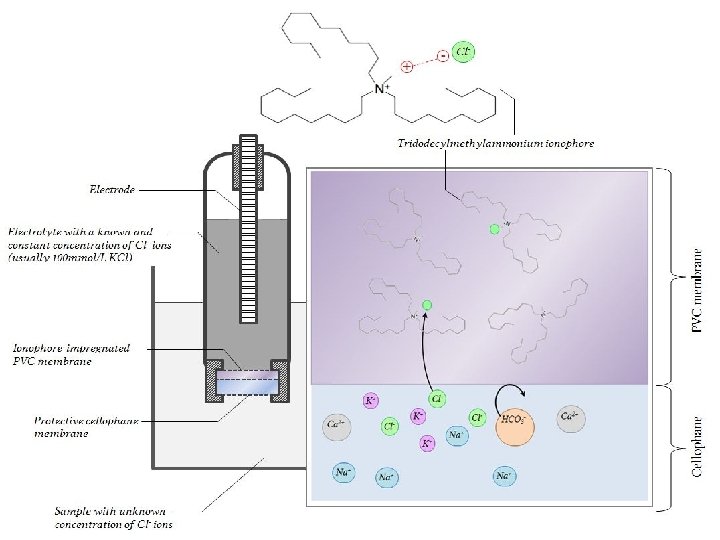
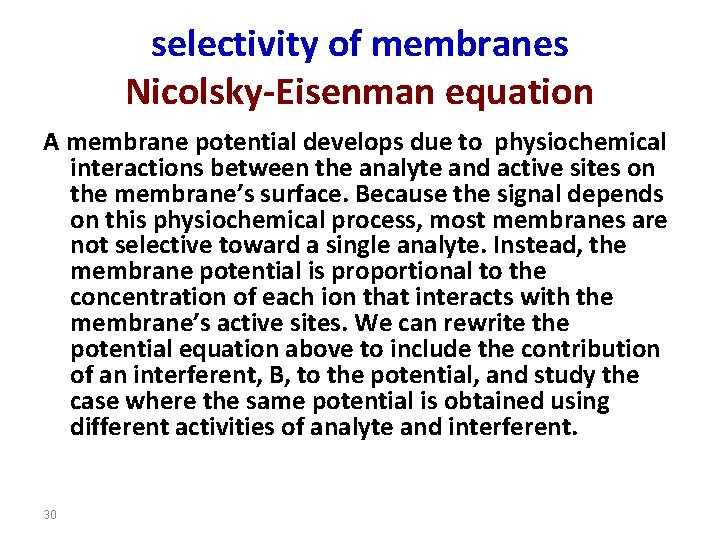
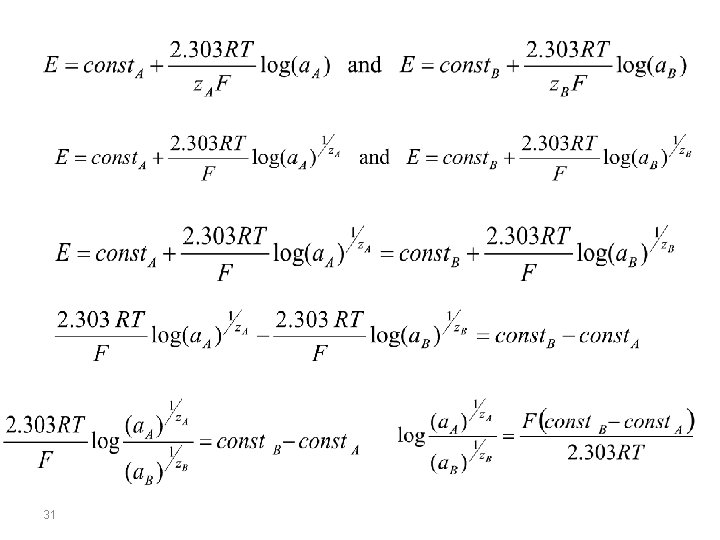
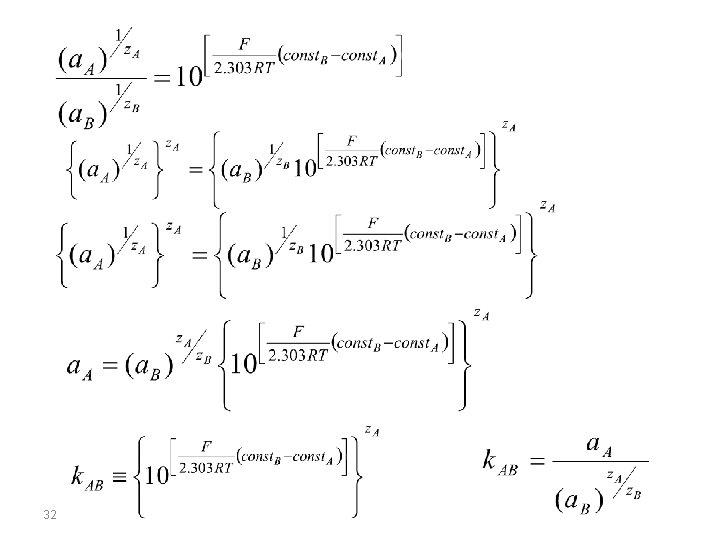
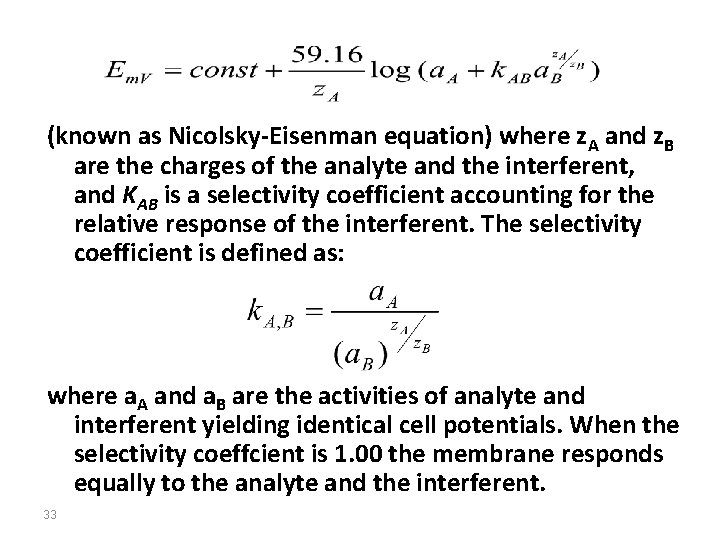
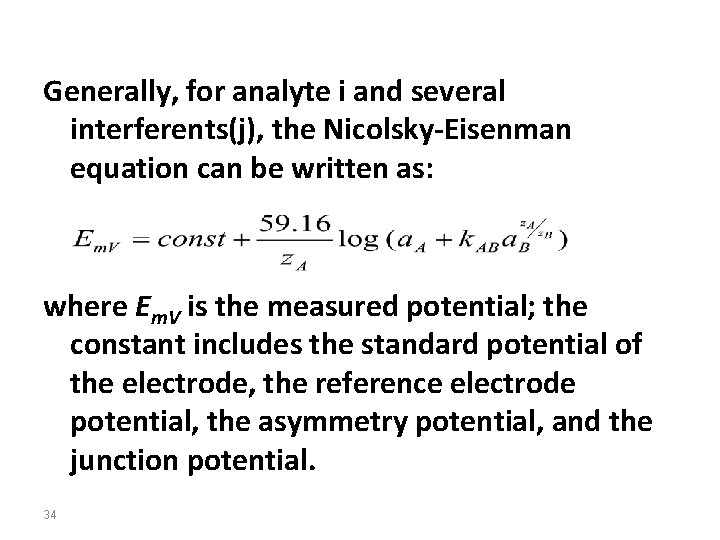
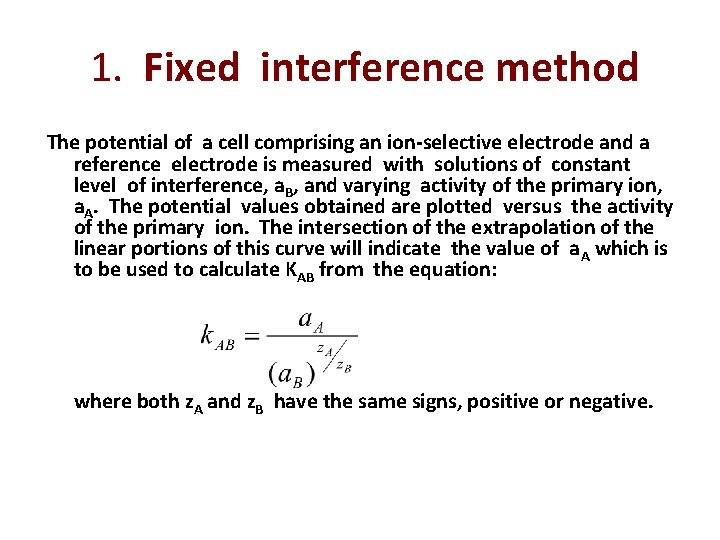
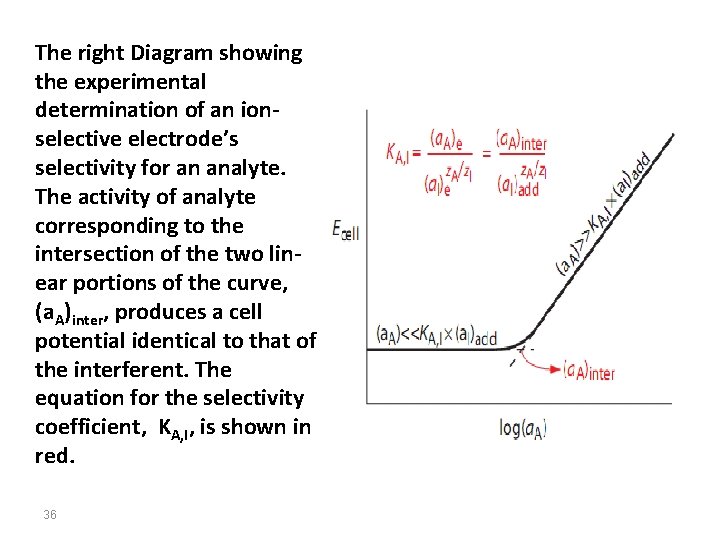
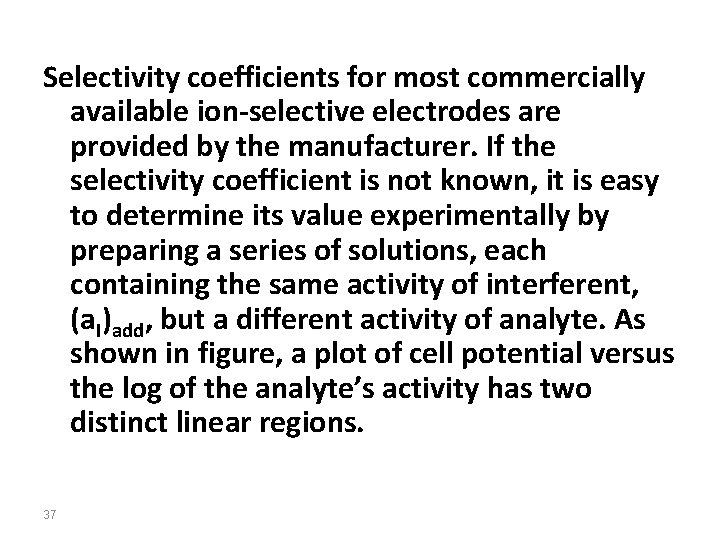
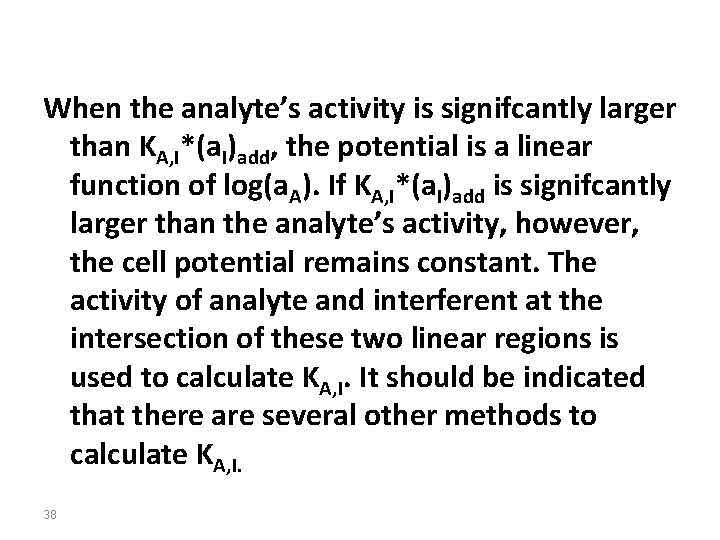
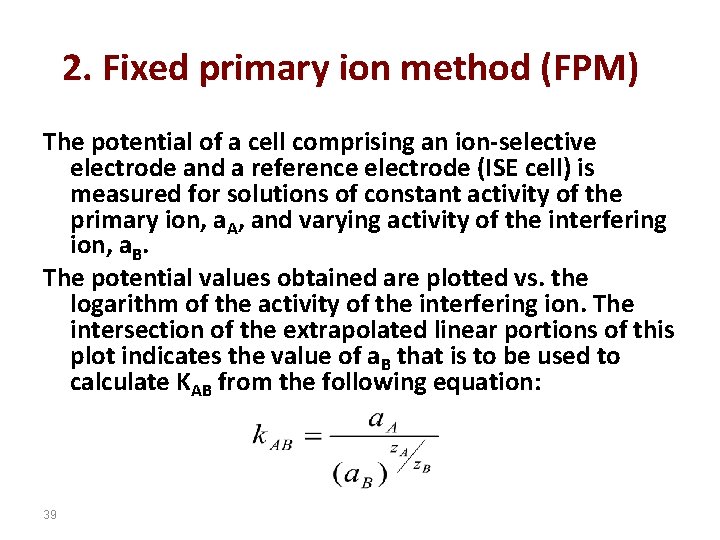
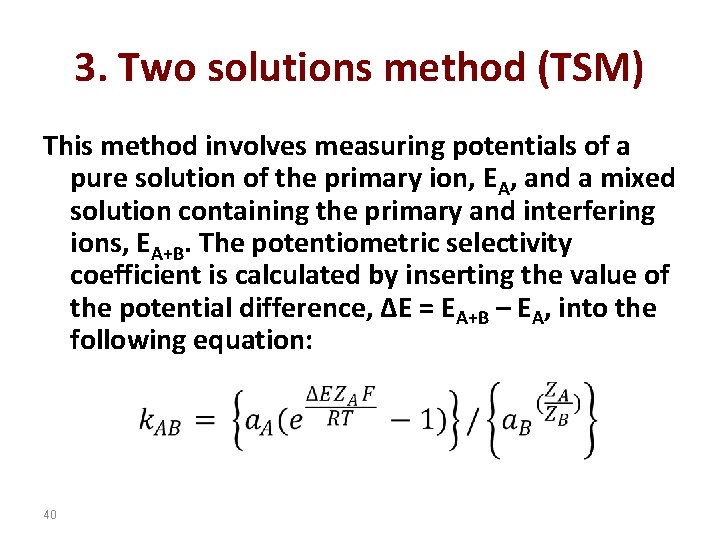
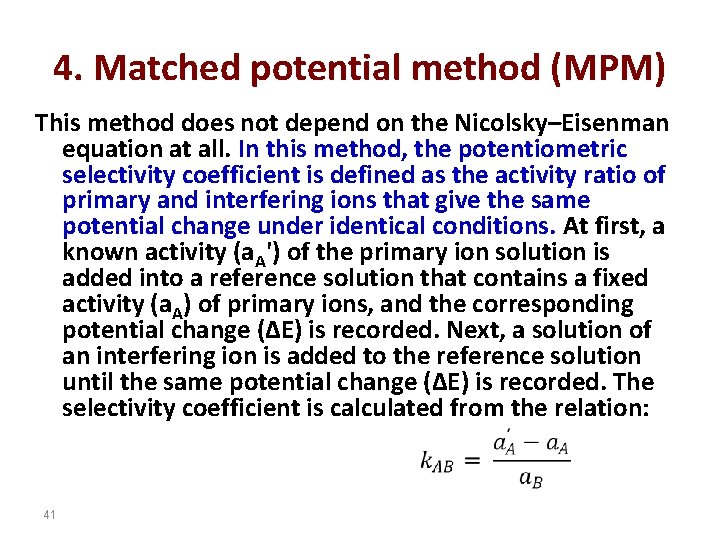
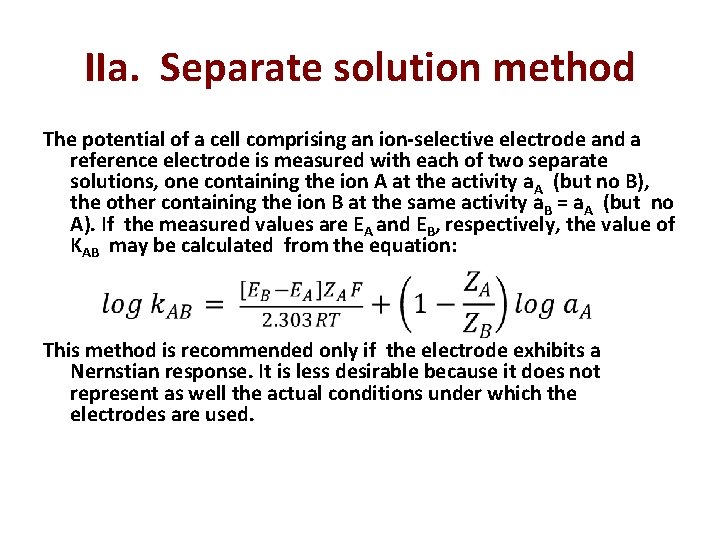
![IIb. Separate solution method (EA = EB) [SSM (EA = EB)] The log a IIb. Separate solution method (EA = EB) [SSM (EA = EB)] The log a](https://slidetodoc.com/presentation_image/3ae7c1ec86985151055d455023484592/image-43.jpg)
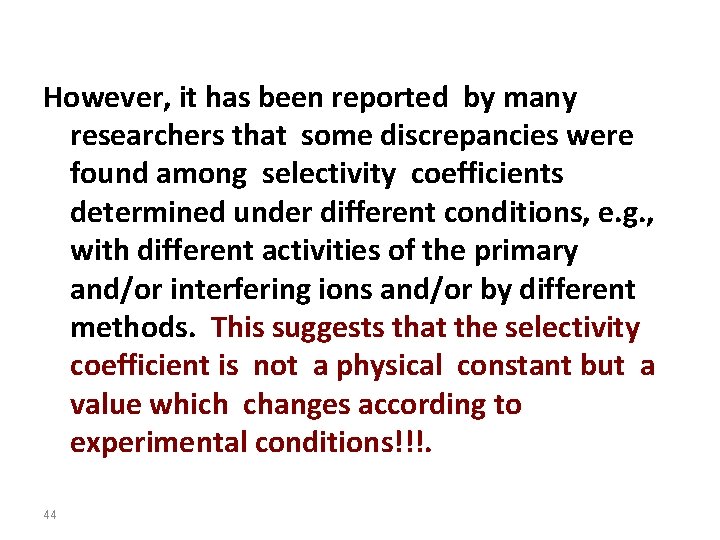
- Slides: 44
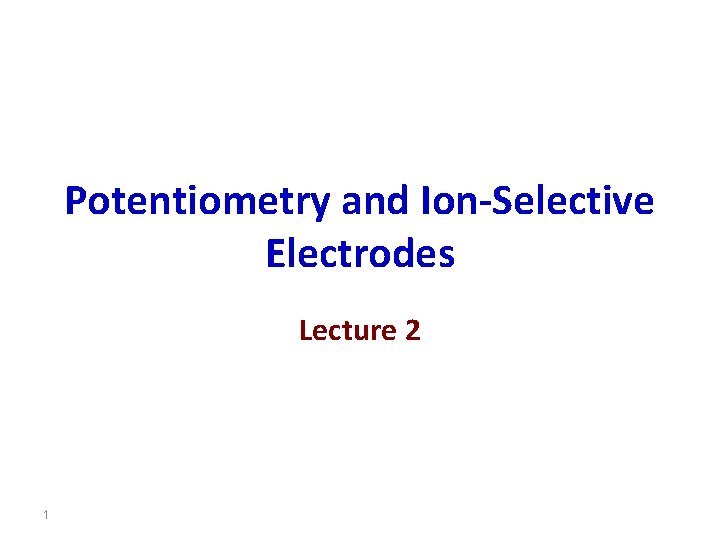
Potentiometry and Ion-Selective Electrodes Lecture 2 1
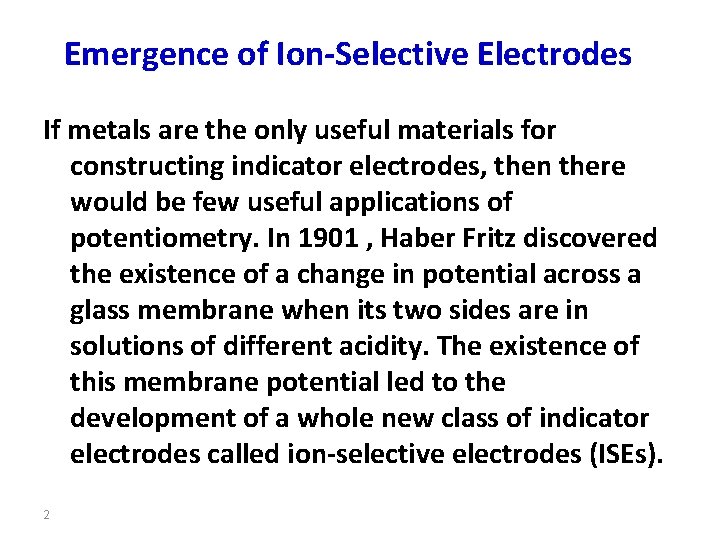
Emergence of Ion-Selective Electrodes If metals are the only useful materials for constructing indicator electrodes, then there would be few useful applications of potentiometry. In 1901 , Haber Fritz discovered the existence of a change in potential across a glass membrane when its two sides are in solutions of different acidity. The existence of this membrane potential led to the development of a whole new class of indicator electrodes called ion-selective electrodes (ISEs). 2
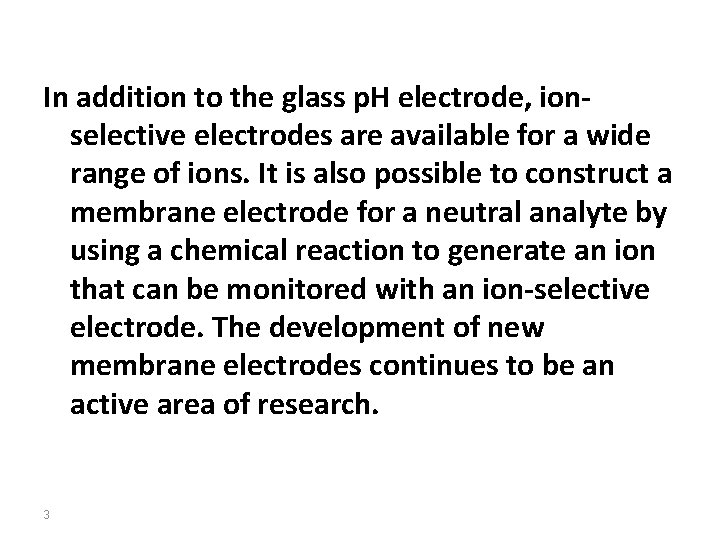
In addition to the glass p. H electrode, ionselective electrodes are available for a wide range of ions. It is also possible to construct a membrane electrode for a neutral analyte by using a chemical reaction to generate an ion that can be monitored with an ion-selective electrode. The development of new membrane electrodes continues to be an active area of research. 3
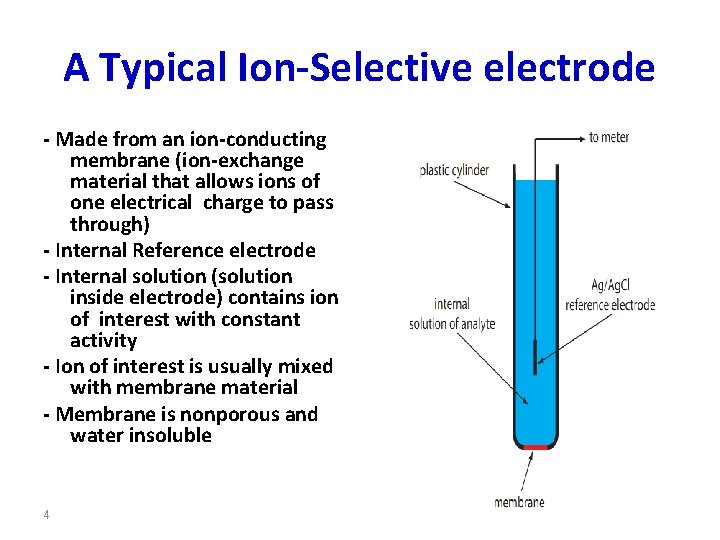
A Typical Ion-Selective electrode - Made from an ion-conducting membrane (ion-exchange material that allows ions of one electrical charge to pass through) - Internal Reference electrode - Internal solution (solution inside electrode) contains ion of interest with constant activity - Ion of interest is usually mixed with membrane material - Membrane is nonporous and water insoluble 4
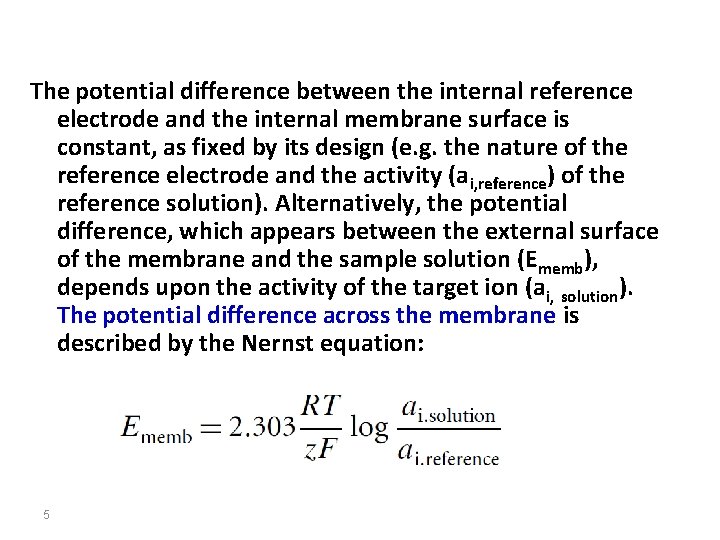
The potential difference between the internal reference electrode and the internal membrane surface is constant, as fixed by its design (e. g. the nature of the reference electrode and the activity (ai, reference) of the reference solution). Alternatively, the potential difference, which appears between the external surface of the membrane and the sample solution (Ememb), depends upon the activity of the target ion (ai, solution). The potential difference across the membrane is described by the Nernst equation: 5
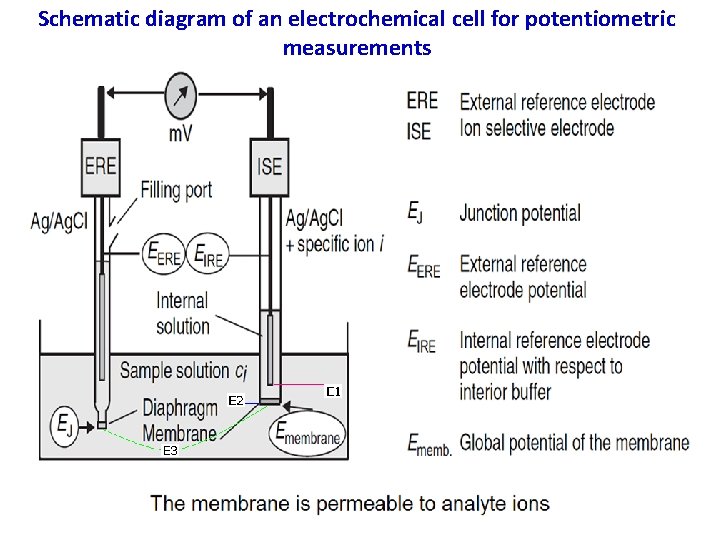
Schematic diagram of an electrochemical cell for potentiometric measurements 6
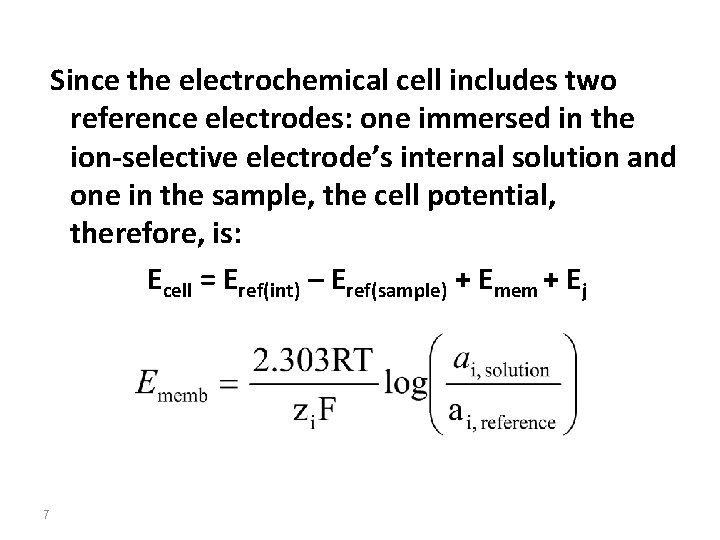
Since the electrochemical cell includes two reference electrodes: one immersed in the ion-selective electrode’s internal solution and one in the sample, the cell potential, therefore, is: Ecell = Eref(int) – Eref(sample) + Emem + Ej 7
![IONSELECTIVE ELECTRODES ISE Analyte ion i inside the electrode analyte ion i ION-SELECTIVE ELECTRODES (ISE) - [Analyte ion, i] inside the electrode ≠ [analyte ion, i]](https://slidetodoc.com/presentation_image/3ae7c1ec86985151055d455023484592/image-8.jpg)
ION-SELECTIVE ELECTRODES (ISE) - [Analyte ion, i] inside the electrode ≠ [analyte ion, i] outside the electrode. - Develop a potential difference across the membrane Generally (at 25 o. C) - 10 -fold change in activity implies 59/zi m. V change in E, for singly charged ions. - zi is the charge on the analyte ion (negative for anions) - zi = +1 for K+, zi = +2 for Ca 2+, zi = -2 for CO 32 - 8
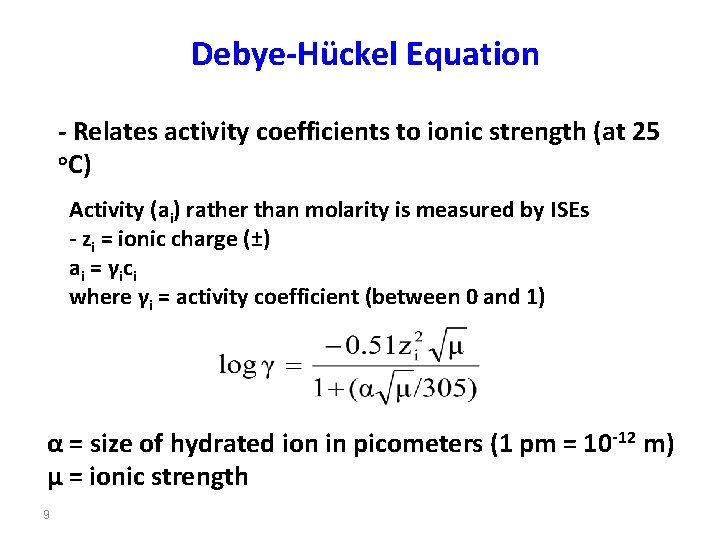
Debye-Hückel Equation - Relates activity coefficients to ionic strength (at 25 o. C) Activity (ai) rather than molarity is measured by ISEs - zi = ionic charge (±) a i = γi c i where γi = activity coefficient (between 0 and 1) α = size of hydrated ion in picometers (1 pm = 10 -12 m) µ = ionic strength 9
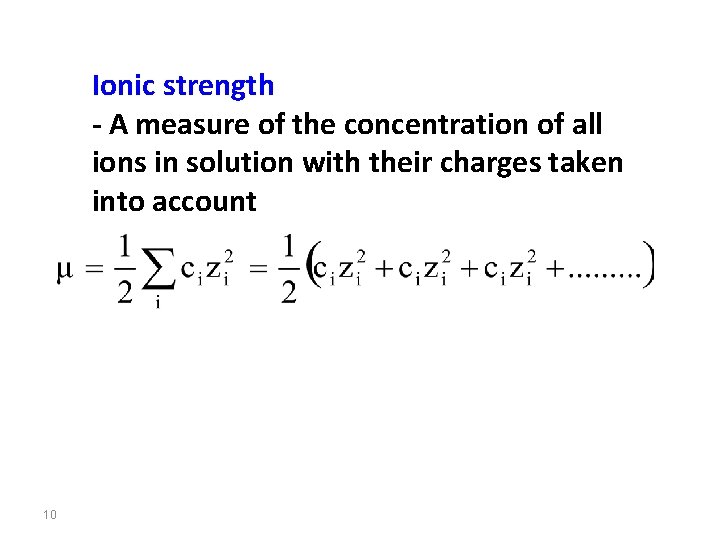
Ionic strength - A measure of the concentration of all ions in solution with their charges taken into account 10
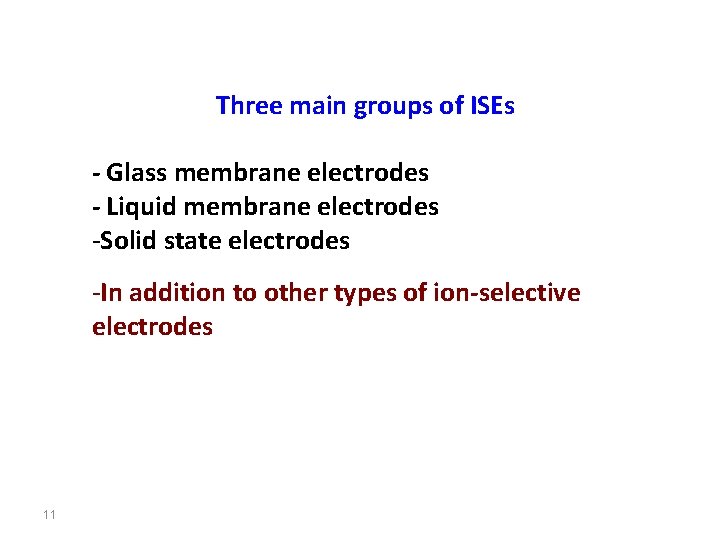
Three main groups of ISEs - Glass membrane electrodes - Liquid membrane electrodes -Solid state electrodes -In addition to other types of ion-selective electrodes 11
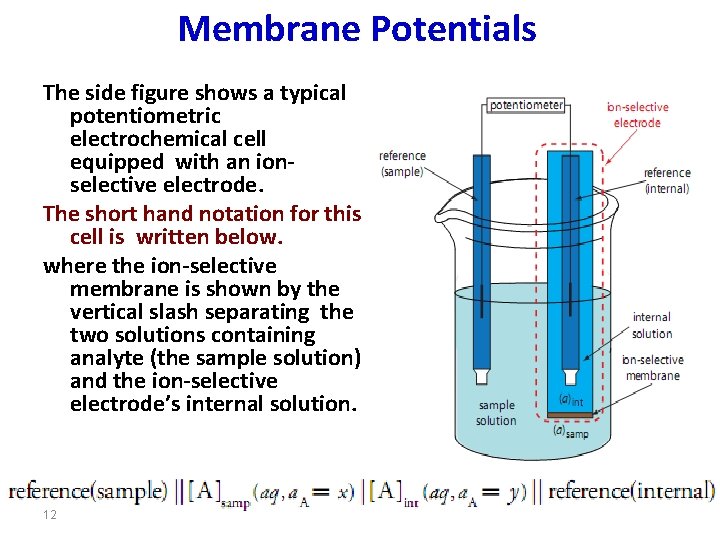
Membrane Potentials The side figure shows a typical potentiometric electrochemical cell equipped with an ionselective electrode. The short hand notation for this cell is written below. where the ion-selective membrane is shown by the vertical slash separating the two solutions containing analyte (the sample solution) and the ion-selective electrode’s internal solution. 12
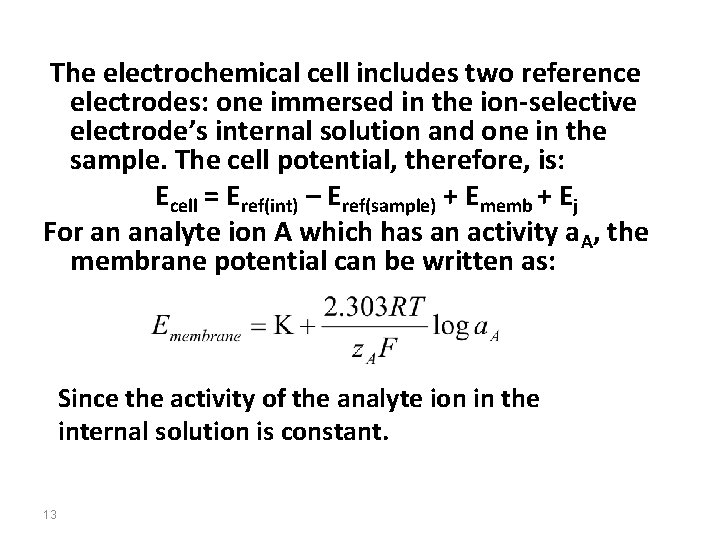
The electrochemical cell includes two reference electrodes: one immersed in the ion-selective electrode’s internal solution and one in the sample. The cell potential, therefore, is: Ecell = Eref(int) – Eref(sample) + Ememb + Ej For an analyte ion A which has an activity a. A, the membrane potential can be written as: Since the activity of the analyte ion in the internal solution is constant. 13
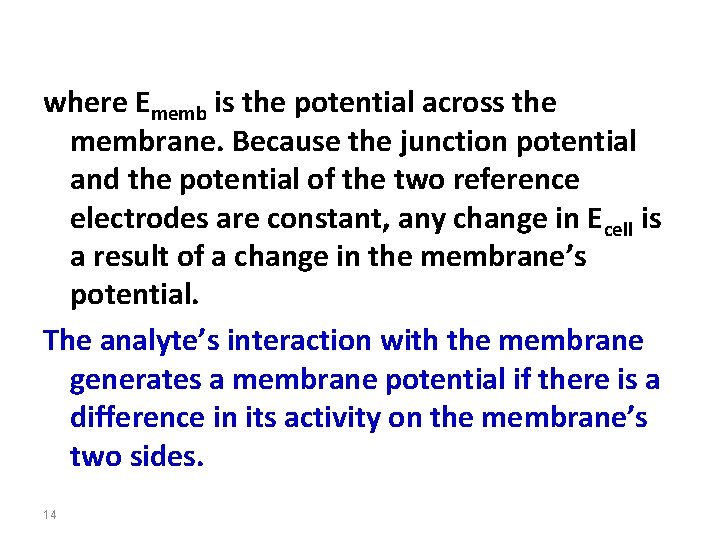
where Ememb is the potential across the membrane. Because the junction potential and the potential of the two reference electrodes are constant, any change in Ecell is a result of a change in the membrane’s potential. The analyte’s interaction with the membrane generates a membrane potential if there is a difference in its activity on the membrane’s two sides. 14
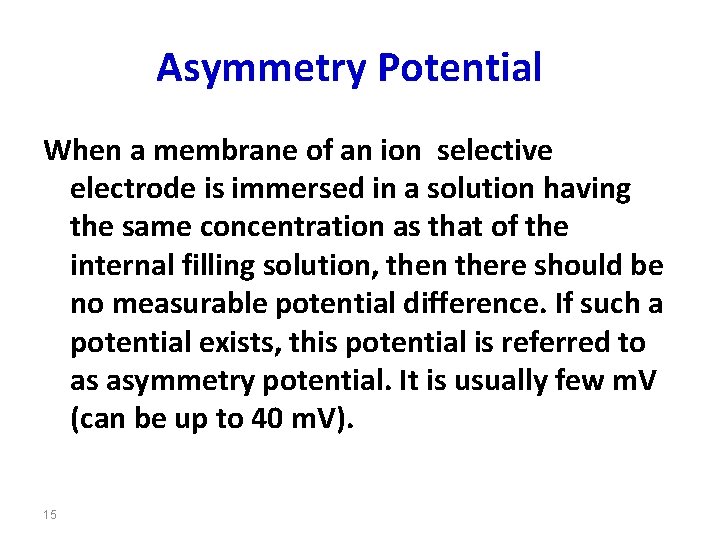
Asymmetry Potential When a membrane of an ion selective electrode is immersed in a solution having the same concentration as that of the internal filling solution, then there should be no measurable potential difference. If such a potential exists, this potential is referred to as asymmetry potential. It is usually few m. V (can be up to 40 m. V). 15
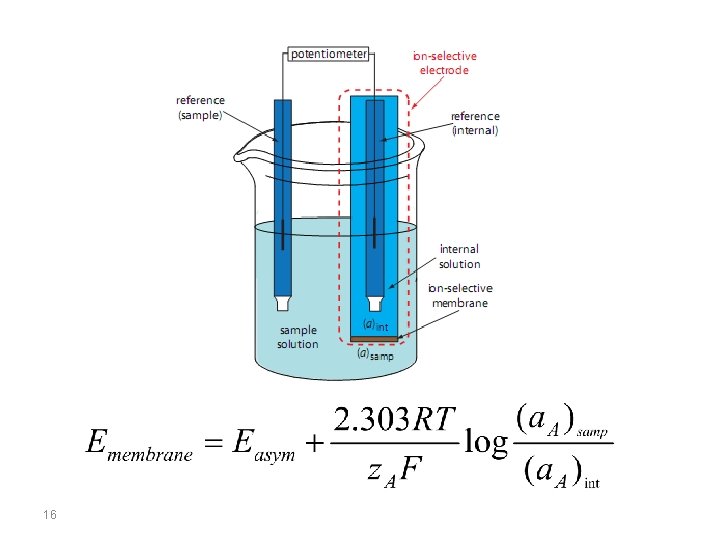
16
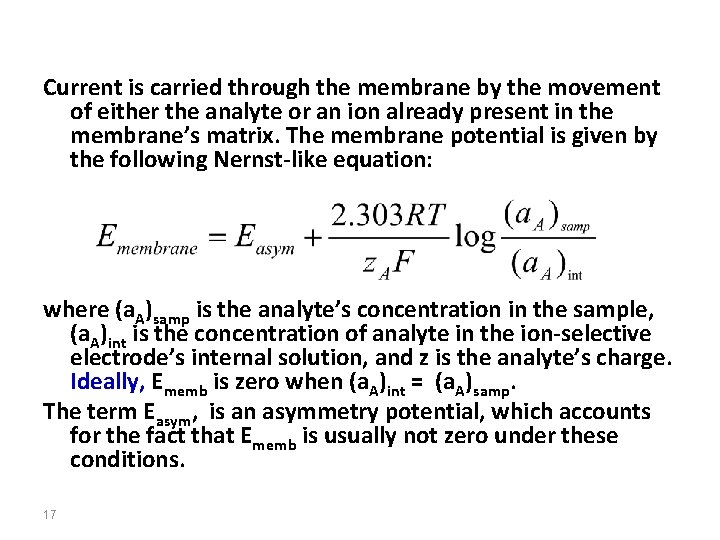
Current is carried through the membrane by the movement of either the analyte or an ion already present in the membrane’s matrix. The membrane potential is given by the following Nernst-like equation: where (a. A)samp is the analyte’s concentration in the sample, (a. A)int is the concentration of analyte in the ion-selective electrode’s internal solution, and z is the analyte’s charge. Ideally, Ememb is zero when (a. A)int = (a. A)samp. The term Easym, is an asymmetry potential, which accounts for the fact that Ememb is usually not zero under these conditions. 17
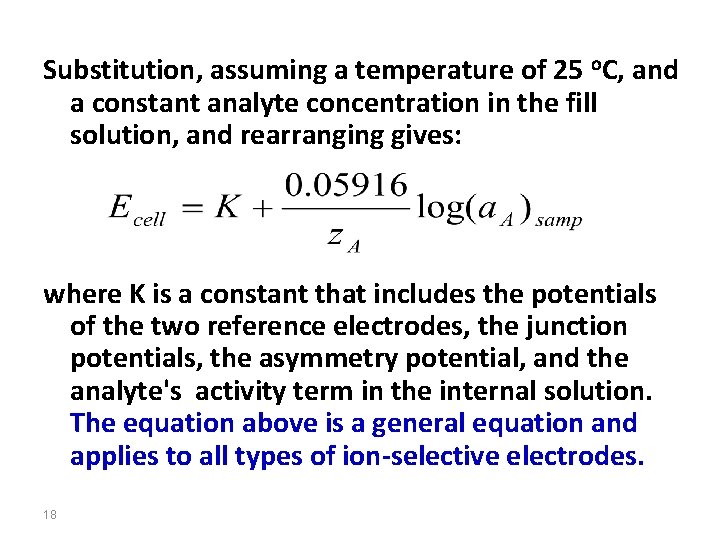
Substitution, assuming a temperature of 25 o. C, and a constant analyte concentration in the fill solution, and rearranging gives: where K is a constant that includes the potentials of the two reference electrodes, the junction potentials, the asymmetry potential, and the analyte's activity term in the internal solution. The equation above is a general equation and applies to all types of ion-selective electrodes. 18
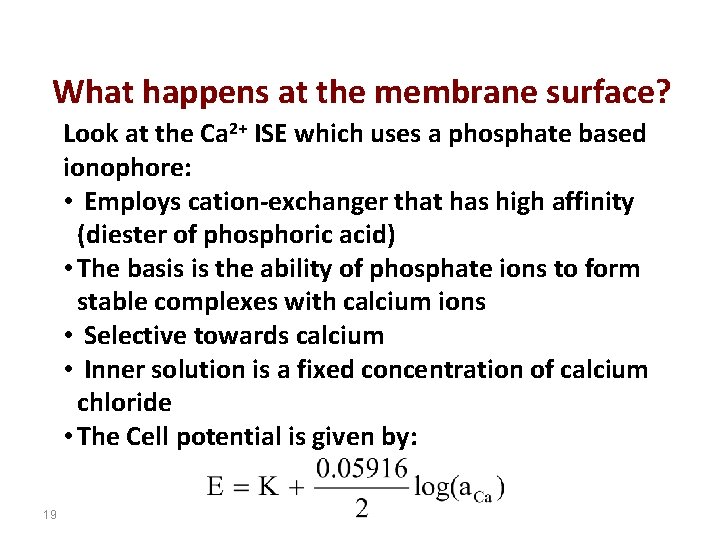
What happens at the membrane surface? Look at the Ca 2+ ISE which uses a phosphate based ionophore: • Employs cation-exchanger that has high affinity (diester of phosphoric acid) • The basis is the ability of phosphate ions to form stable complexes with calcium ions • Selective towards calcium • Inner solution is a fixed concentration of calcium chloride • The Cell potential is given by: 19
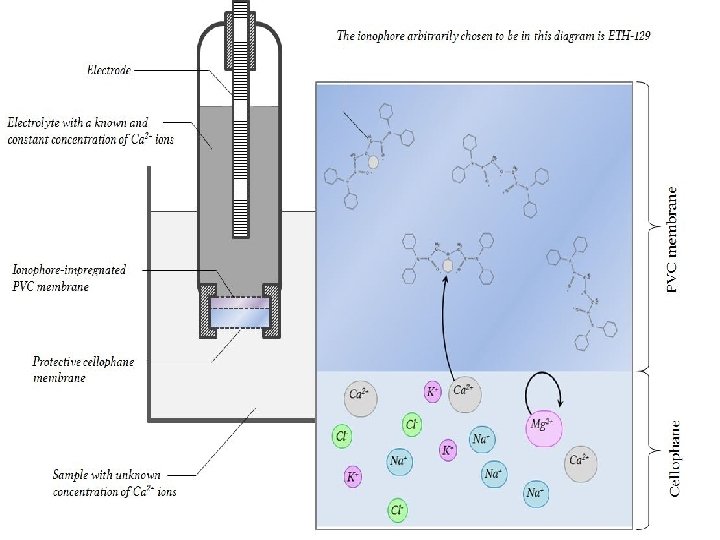
20
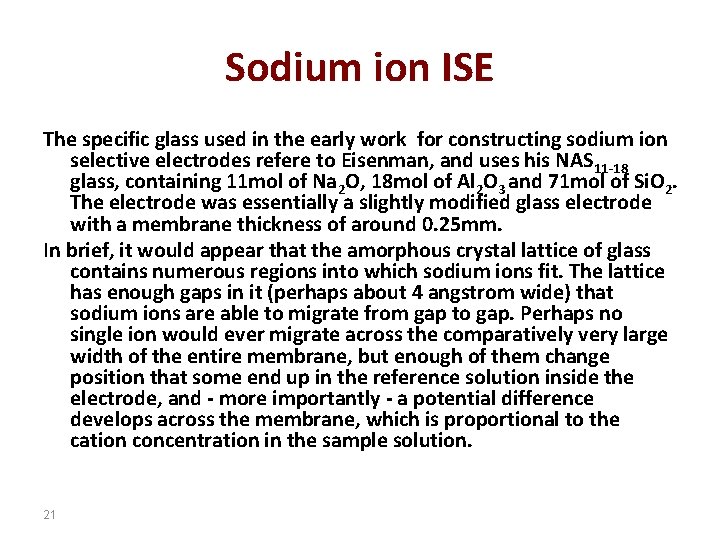
Sodium ion ISE The specific glass used in the early work for constructing sodium ion selective electrodes refere to Eisenman, and uses his NAS 11 -18 glass, containing 11 mol of Na 2 O, 18 mol of Al 2 O 3 and 71 mol of Si. O 2. The electrode was essentially a slightly modified glass electrode with a membrane thickness of around 0. 25 mm. In brief, it would appear that the amorphous crystal lattice of glass contains numerous regions into which sodium ions fit. The lattice has enough gaps in it (perhaps about 4 angstrom wide) that sodium ions are able to migrate from gap to gap. Perhaps no single ion would ever migrate across the comparatively very large width of the entire membrane, but enough of them change position that some end up in the reference solution inside the electrode, and - more importantly - a potential difference develops across the membrane, which is proportional to the cation concentration in the sample solution. 21
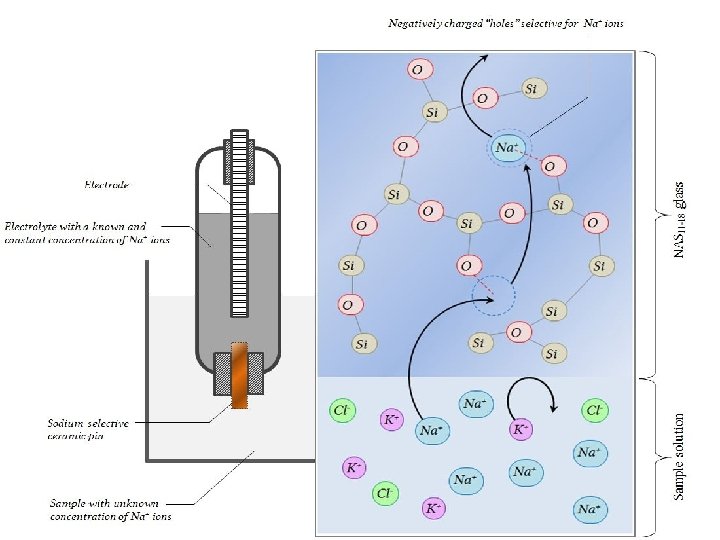
22
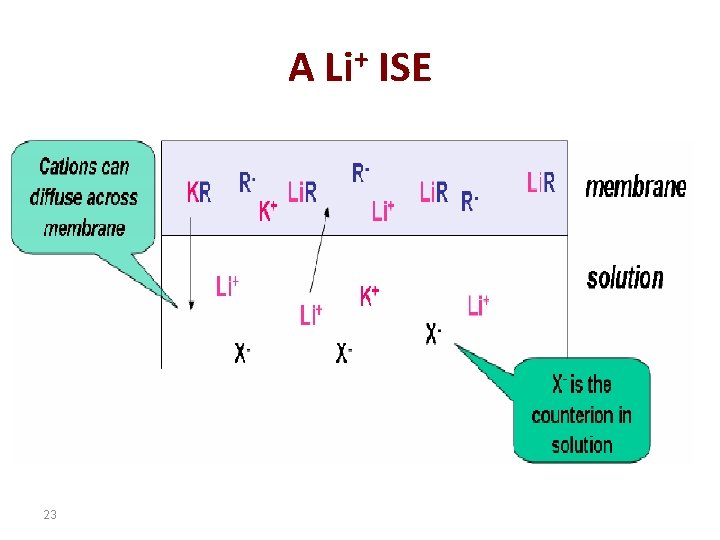
A Li+ ISE 23
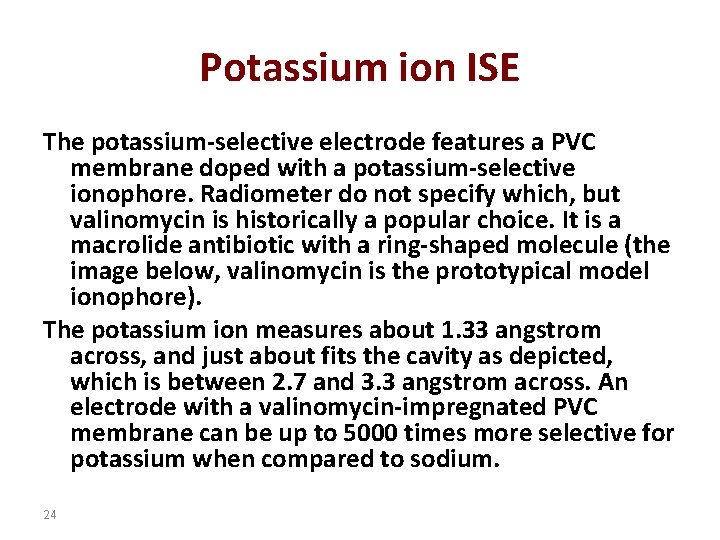
Potassium ion ISE The potassium-selective electrode features a PVC membrane doped with a potassium-selective ionophore. Radiometer do not specify which, but valinomycin is historically a popular choice. It is a macrolide antibiotic with a ring-shaped molecule (the image below, valinomycin is the prototypical model ionophore). The potassium ion measures about 1. 33 angstrom across, and just about fits the cavity as depicted, which is between 2. 7 and 3. 3 angstrom across. An electrode with a valinomycin-impregnated PVC membrane can be up to 5000 times more selective for potassium when compared to sodium. 24
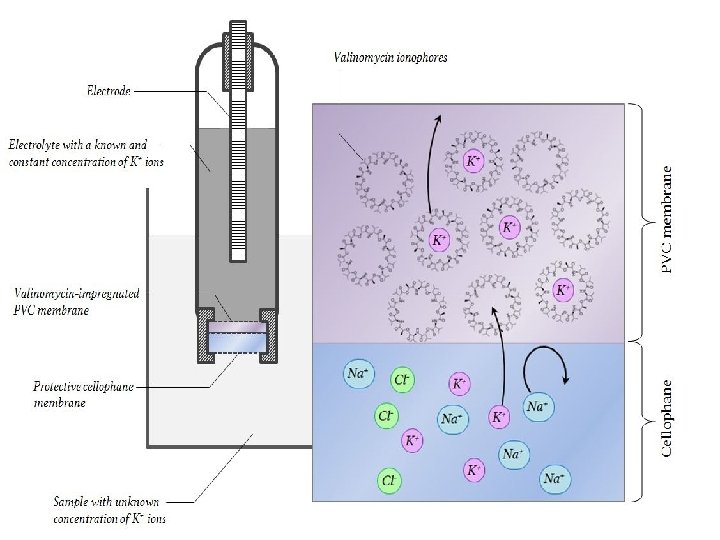
25
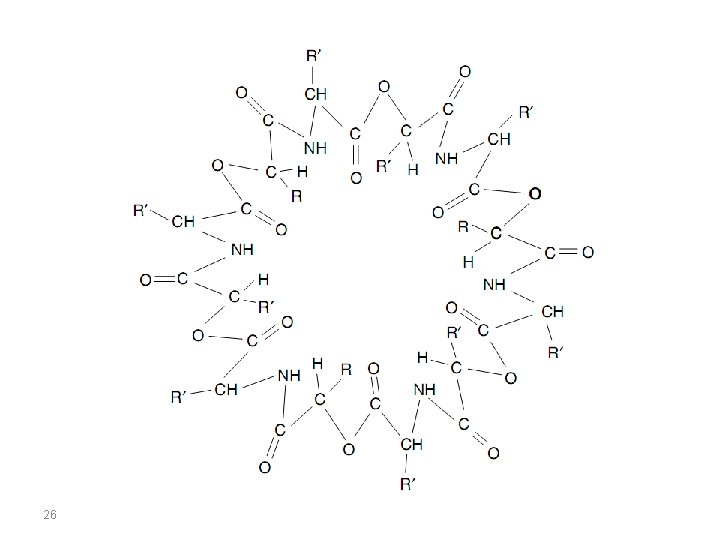
26
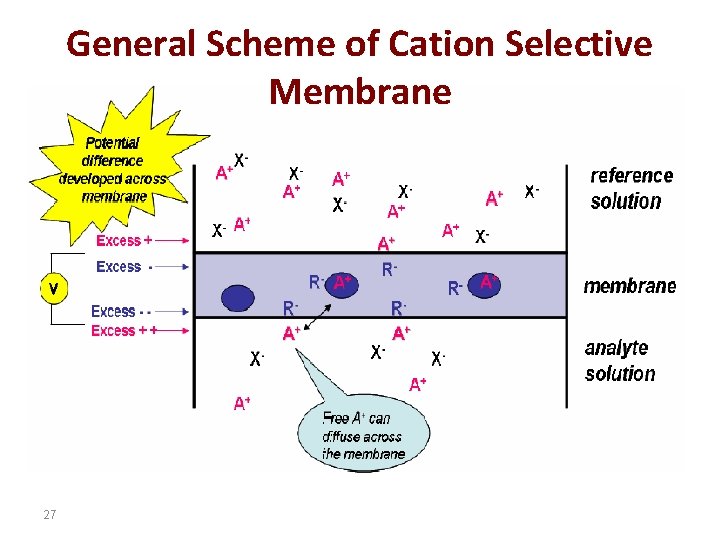
General Scheme of Cation Selective Membrane 27
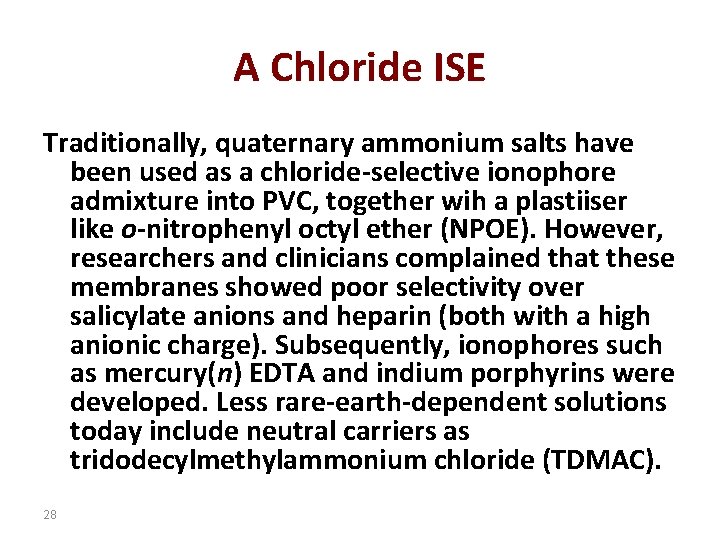
A Chloride ISE Traditionally, quaternary ammonium salts have been used as a chloride-selective ionophore admixture into PVC, together wih a plastiiser like o-nitrophenyl octyl ether (NPOE). However, researchers and clinicians complained that these membranes showed poor selectivity over salicylate anions and heparin (both with a high anionic charge). Subsequently, ionophores such as mercury(n) EDTA and indium porphyrins were developed. Less rare-earth-dependent solutions today include neutral carriers as tridodecylmethylammonium chloride (TDMAC). 28
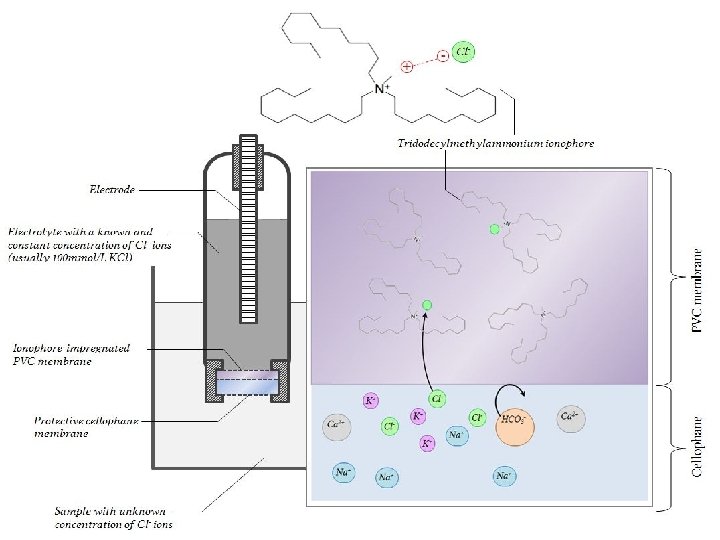
29
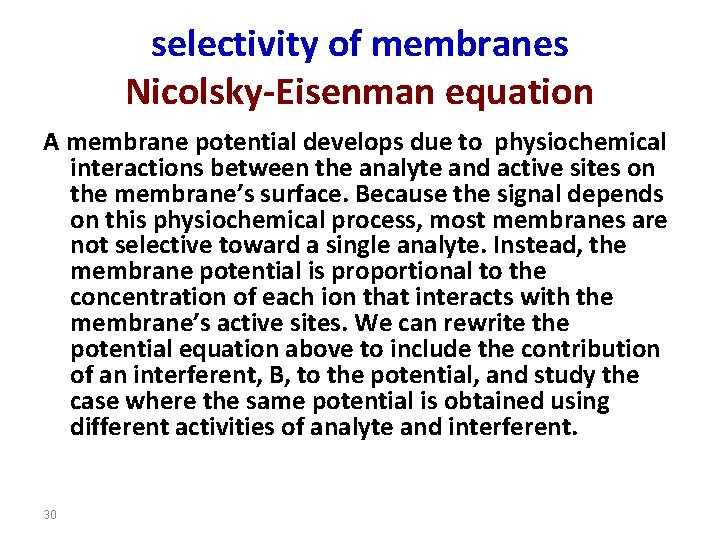
selectivity of membranes Nicolsky-Eisenman equation A membrane potential develops due to physiochemical interactions between the analyte and active sites on the membrane’s surface. Because the signal depends on this physiochemical process, most membranes are not selective toward a single analyte. Instead, the membrane potential is proportional to the concentration of each ion that interacts with the membrane’s active sites. We can rewrite the potential equation above to include the contribution of an interferent, B, to the potential, and study the case where the same potential is obtained using different activities of analyte and interferent. 30
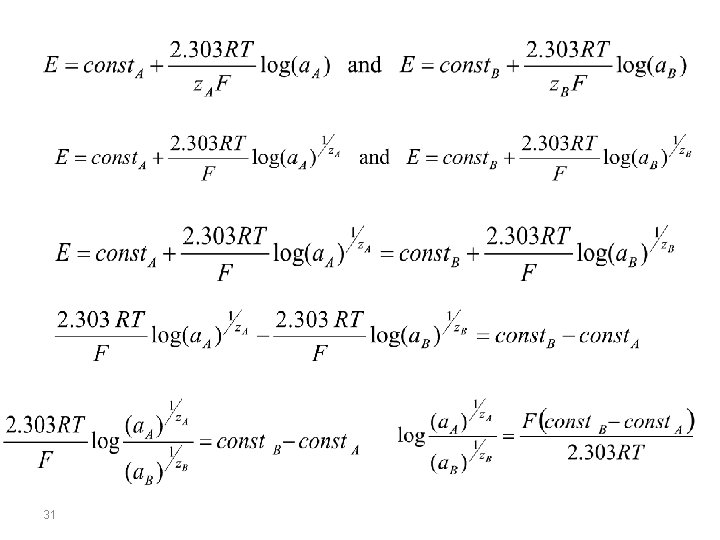
31
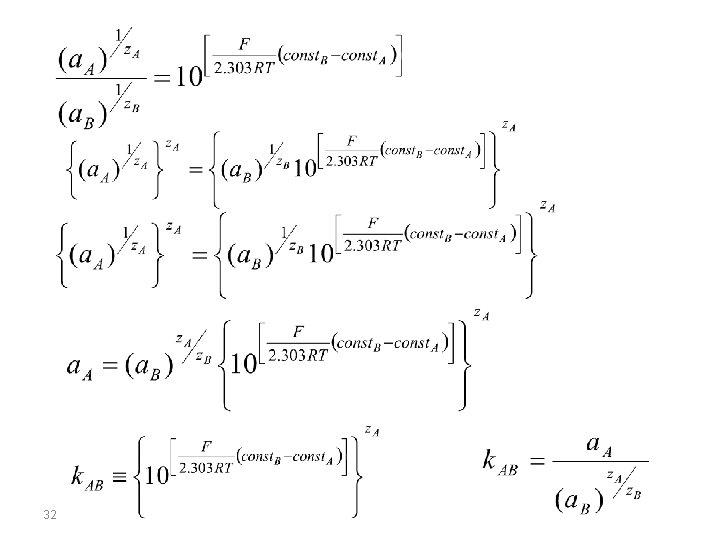
32
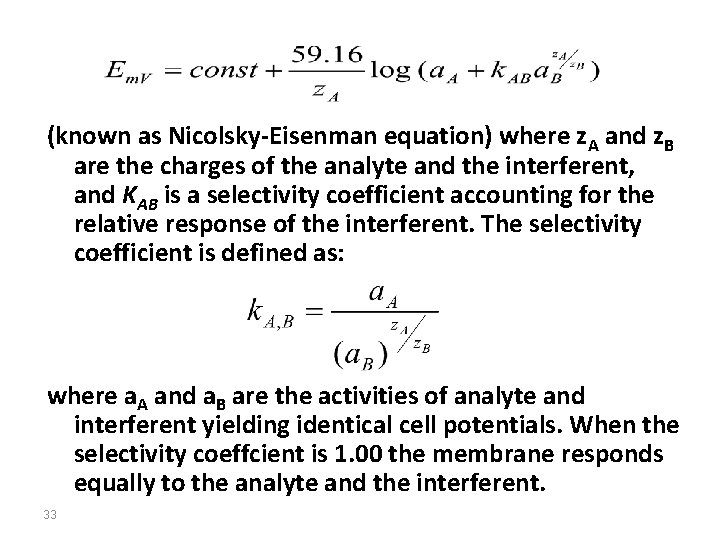
(known as Nicolsky-Eisenman equation) where z. A and z. B are the charges of the analyte and the interferent, and KAB is a selectivity coefficient accounting for the relative response of the interferent. The selectivity coefficient is defined as: where a. A and a. B are the activities of analyte and interferent yielding identical cell potentials. When the selectivity coeffcient is 1. 00 the membrane responds equally to the analyte and the interferent. 33
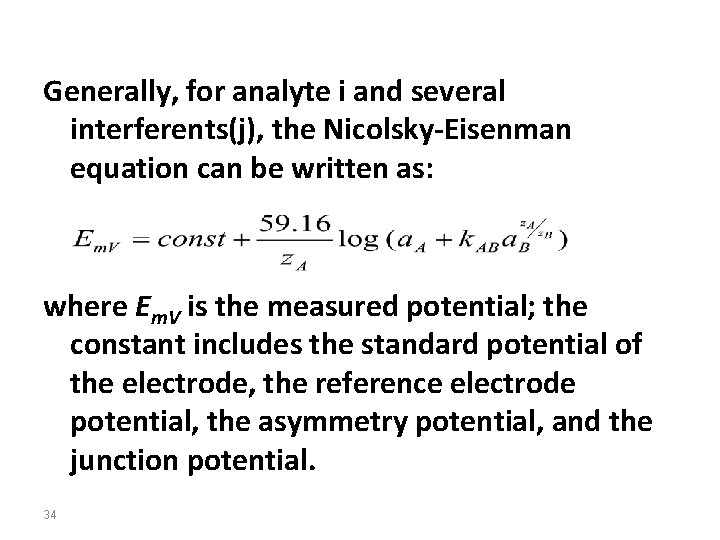
Generally, for analyte i and several interferents(j), the Nicolsky-Eisenman equation can be written as: where Em. V is the measured potential; the constant includes the standard potential of the electrode, the reference electrode potential, the asymmetry potential, and the junction potential. 34
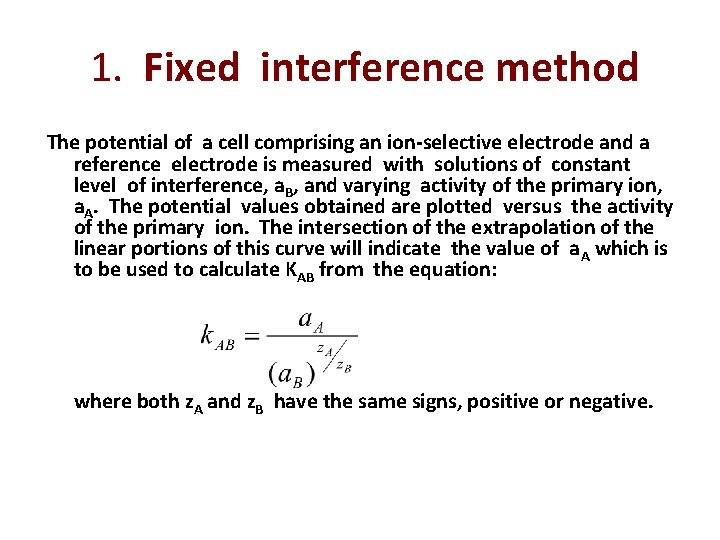
1. Fixed interference method The potential of a cell comprising an ion-selective electrode and a reference electrode is measured with solutions of constant level of interference, a. B, and varying activity of the primary ion, a. A. The potential values obtained are plotted versus the activity of the primary ion. The intersection of the extrapolation of the linear portions of this curve will indicate the value of a. A which is to be used to calculate KAB from the equation: where both z. A and z. B have the same signs, positive or negative.
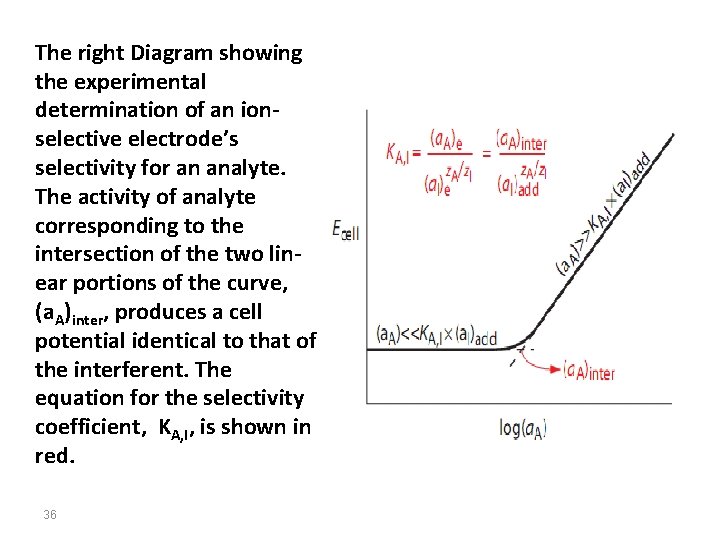
The right Diagram showing the experimental determination of an ionselective electrode’s selectivity for an analyte. The activity of analyte corresponding to the intersection of the two linear portions of the curve, (a. A)inter, produces a cell potential identical to that of the interferent. The equation for the selectivity coefficient, KA, I, is shown in red. 36
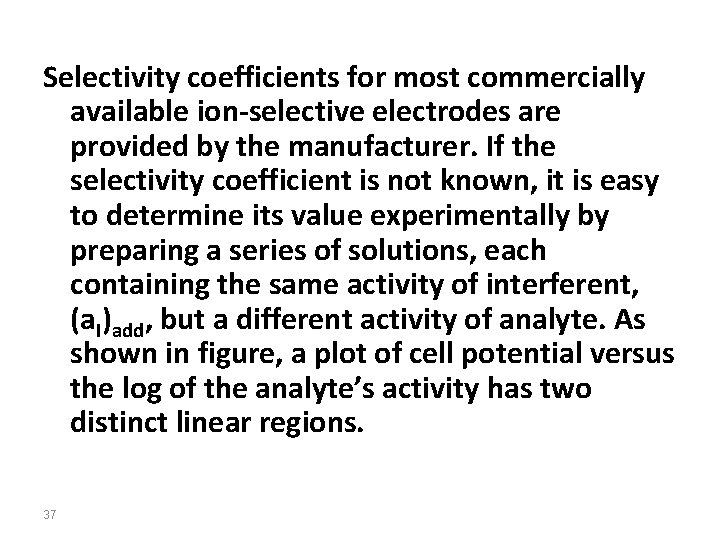
Selectivity coefficients for most commercially available ion-selective electrodes are provided by the manufacturer. If the selectivity coefficient is not known, it is easy to determine its value experimentally by preparing a series of solutions, each containing the same activity of interferent, (a. I)add, but a different activity of analyte. As shown in figure, a plot of cell potential versus the log of the analyte’s activity has two distinct linear regions. 37
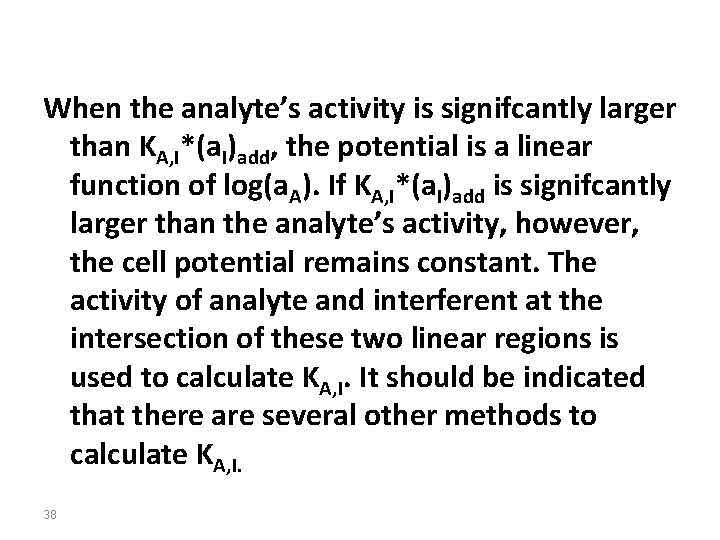
When the analyte’s activity is signifcantly larger than KA, I*(a. I)add, the potential is a linear function of log(a. A). If KA, I*(a. I)add is signifcantly larger than the analyte’s activity, however, the cell potential remains constant. The activity of analyte and interferent at the intersection of these two linear regions is used to calculate KA, I. It should be indicated that there are several other methods to calculate KA, I. 38
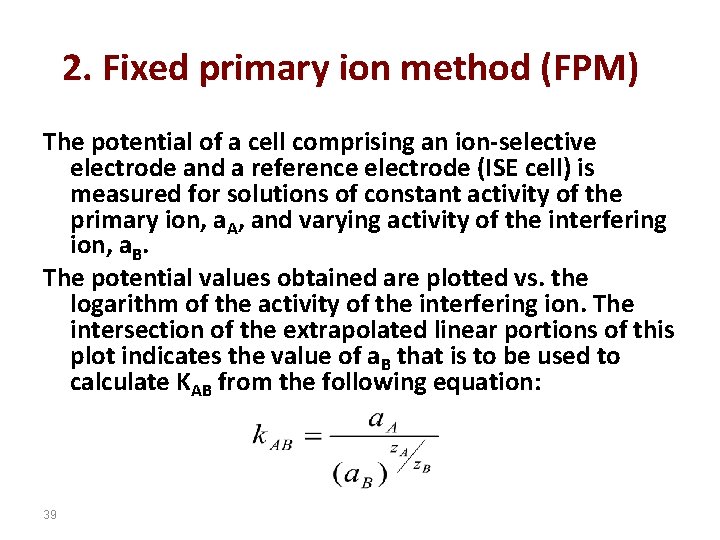
2. Fixed primary ion method (FPM) The potential of a cell comprising an ion-selective electrode and a reference electrode (ISE cell) is measured for solutions of constant activity of the primary ion, a. A, and varying activity of the interfering ion, a. B. The potential values obtained are plotted vs. the logarithm of the activity of the interfering ion. The intersection of the extrapolated linear portions of this plot indicates the value of a. B that is to be used to calculate KAB from the following equation: 39
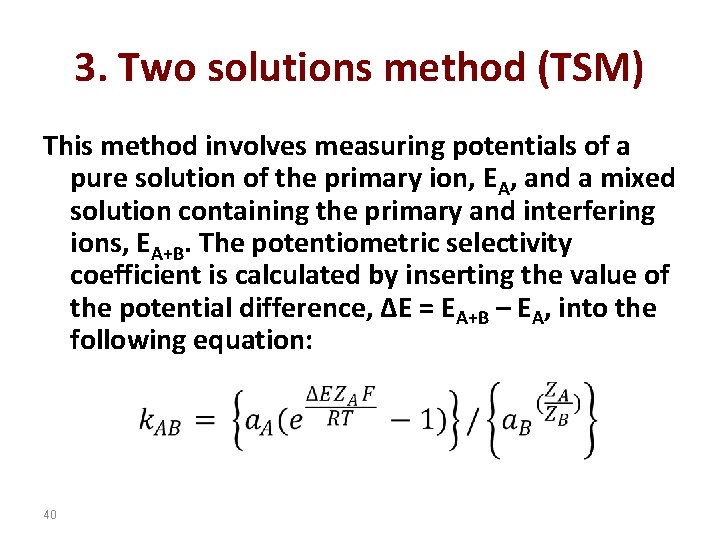
3. Two solutions method (TSM) This method involves measuring potentials of a pure solution of the primary ion, EA, and a mixed solution containing the primary and interfering ions, EA+B. The potentiometric selectivity coefficient is calculated by inserting the value of the potential difference, ∆E = EA+B – EA, into the following equation: 40
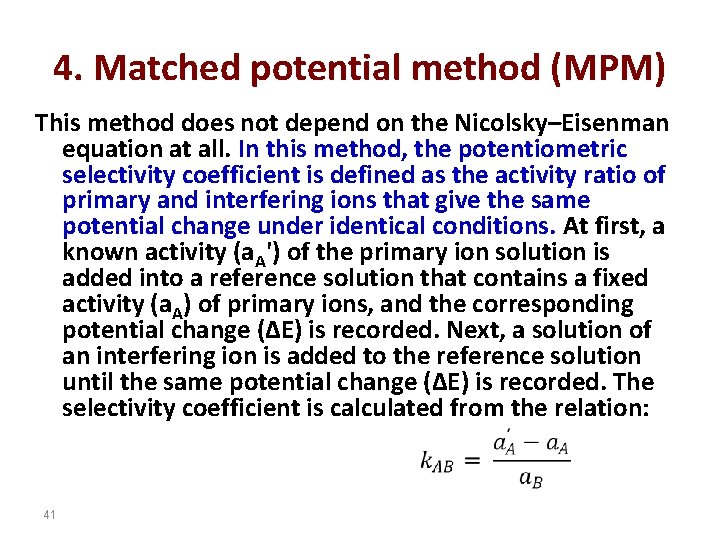
4. Matched potential method (MPM) This method does not depend on the Nicolsky–Eisenman equation at all. In this method, the potentiometric selectivity coefficient is defined as the activity ratio of primary and interfering ions that give the same potential change under identical conditions. At first, a known activity (a. A') of the primary ion solution is added into a reference solution that contains a fixed activity (a. A) of primary ions, and the corresponding potential change (∆E) is recorded. Next, a solution of an interfering ion is added to the reference solution until the same potential change (∆E) is recorded. The selectivity coefficient is calculated from the relation: 41
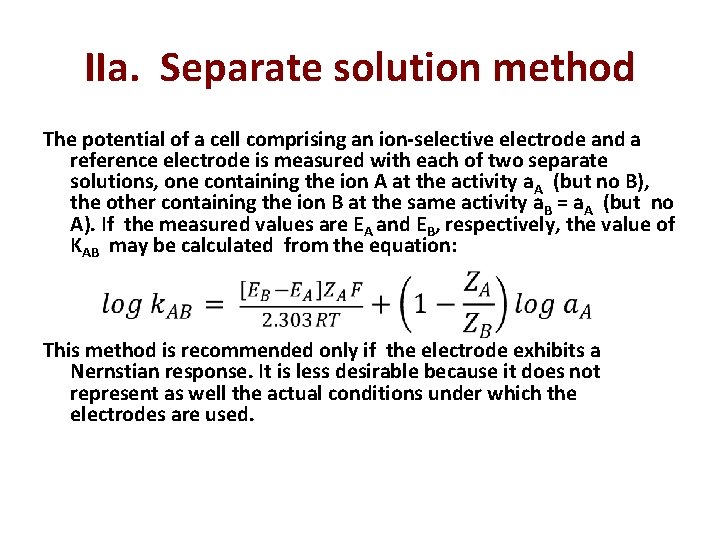
IIa. Separate solution method The potential of a cell comprising an ion-selective electrode and a reference electrode is measured with each of two separate solutions, one containing the ion A at the activity a. A (but no B), the other containing the ion B at the same activity a. B = a. A (but no A). If the measured values are EA and EB, respectively, the value of KAB may be calculated from the equation: This method is recommended only if the electrode exhibits a Nernstian response. It is less desirable because it does not represent as well the actual conditions under which the electrodes are used.
![IIb Separate solution method EA EB SSM EA EB The log a IIb. Separate solution method (EA = EB) [SSM (EA = EB)] The log a](https://slidetodoc.com/presentation_image/3ae7c1ec86985151055d455023484592/image-43.jpg)
IIb. Separate solution method (EA = EB) [SSM (EA = EB)] The log a vs E relation of an ISE for the primary and interfering ions are obtained independently. Then, the activities that correspond to the same electrode potential value are used to determine the KAB value. 43
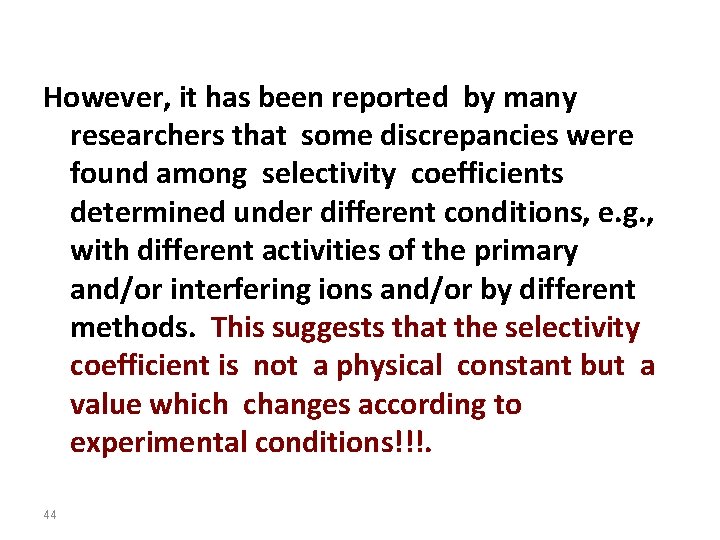
However, it has been reported by many researchers that some discrepancies were found among selectivity coefficients determined under different conditions, e. g. , with different activities of the primary and/or interfering ions and/or by different methods. This suggests that the selectivity coefficient is not a physical constant but a value which changes according to experimental conditions!!!. 44
Types of indicator electrode in potentiometry
Biopotential electrodes lecture notes
Difference between conductometry and potentiometry
Dropping mercury electrode diagram
Difference between voltammetry and potentiometry
Which cell is used in potentiometry
Asymmetry potential
Voltammetry vs amperometry
Reference electrode in potentiometry
Voltammetry and amperometry
Potentiometry
Potentiometric titration
Instrumentation of voltammetry
01:640:244 lecture notes - lecture 15: plat, idah, farad
So&e
Emergence theory
Lesson 2 the emergence of mass society
Systemic game design
Stages of second language development
Emergence of scaling in random networks
Speech emergence stage
Plan emergence madagascar 2019-2023 pdf
Emergence netflix
Entrepreneurship during post independence
The emergence of new values
Plan emergence madagascar 2019-2023 pdf
Itero göteborg
Paul ruthman committee
The emergence of mass society
Fast fill electrodes
Electrolysis of concentrated sulfuric acid
Spoon shaped electrode
Welsh cup electrodes have
Faradaic vs non-faradaic
Capacitive field diathermy
What is surgical diathermy
Fill freeze electrode examples
Floating electrodes for ecg
The fraction of total current carried by an ion is called
Polarizable and non polarizable electrode
Transducers used in biomedical instrumentation
Aps therapy user manual
The two aws classifications for fcaw electrodes are
Ecg technique
Esp electrodes