IAEA Post Graduate Educational Course Radiation Protection and
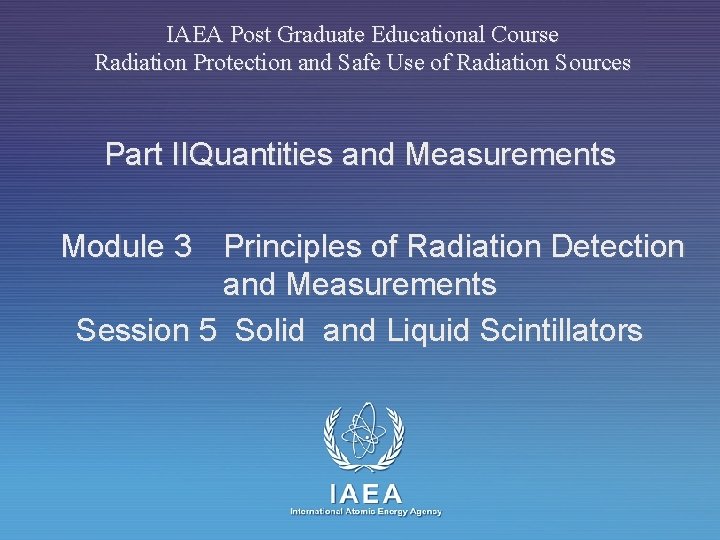
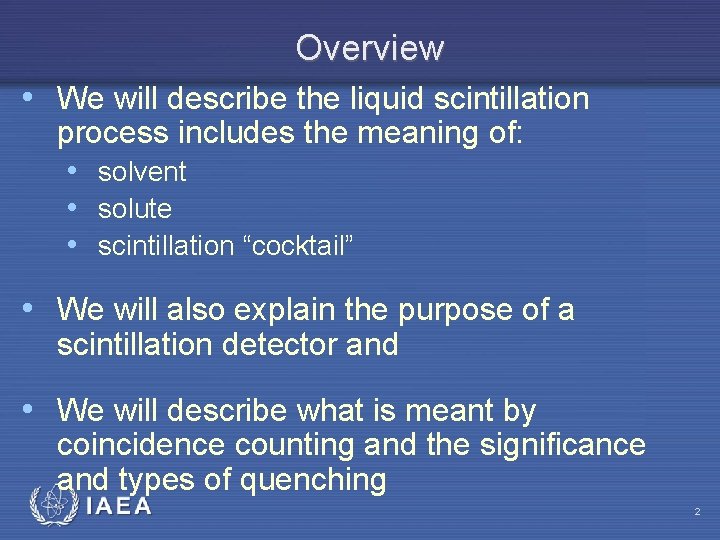
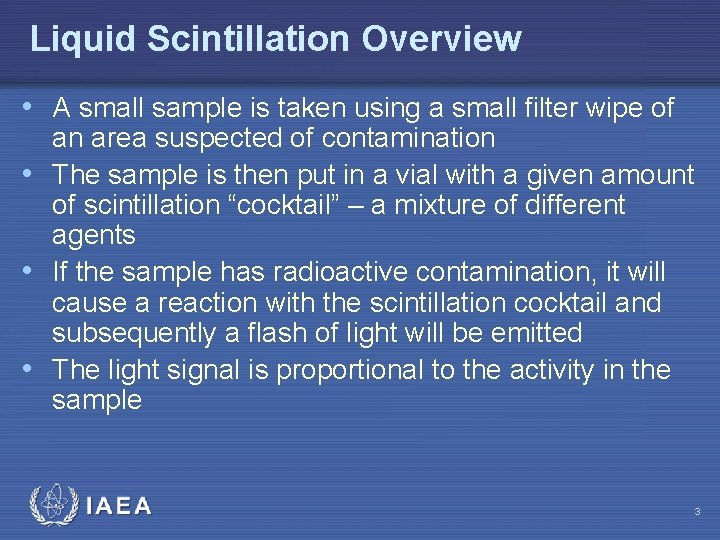
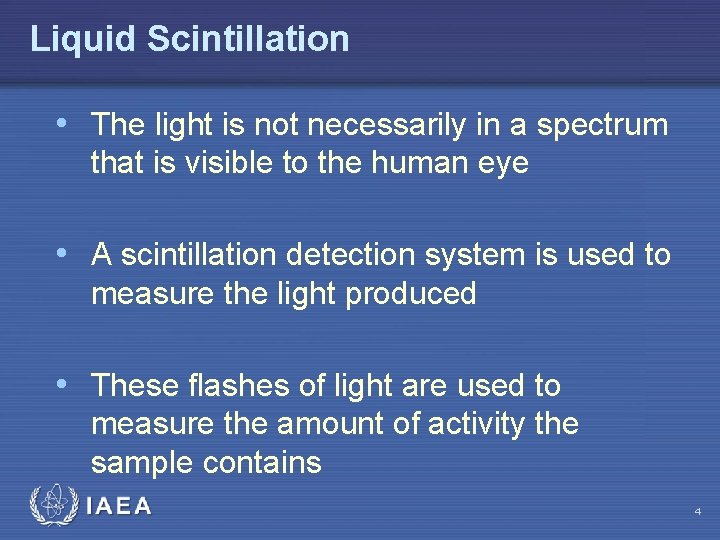
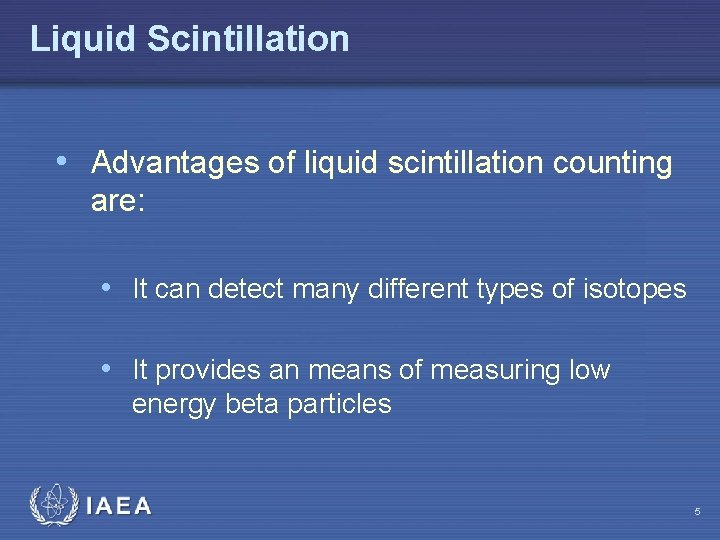
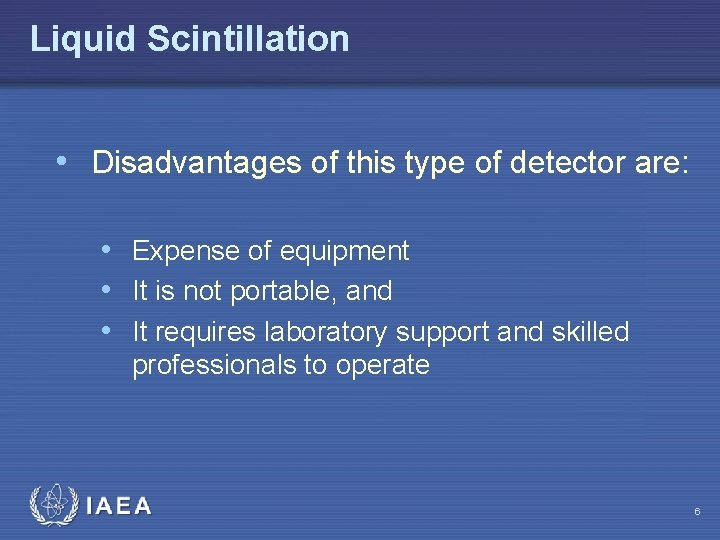
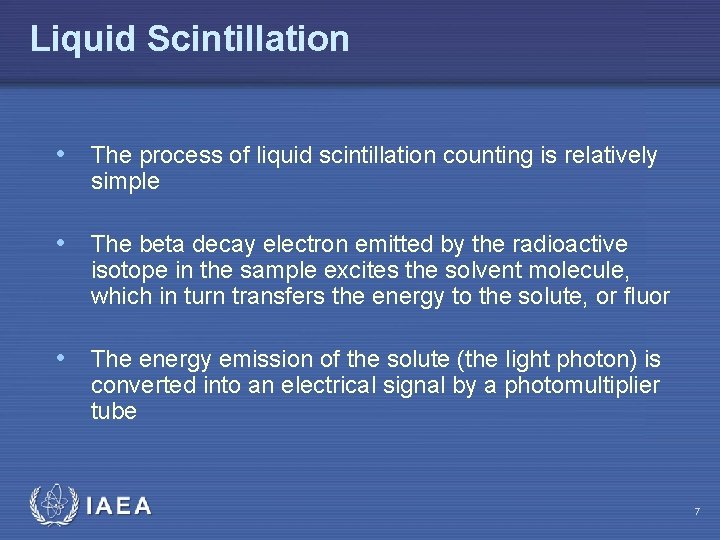
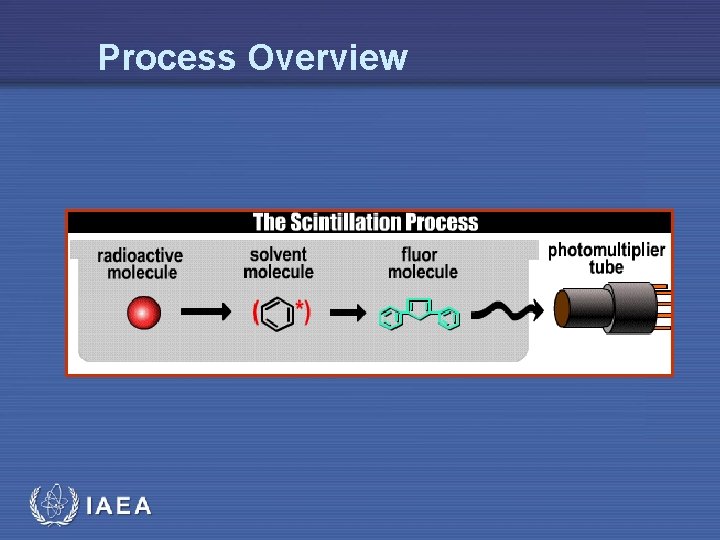
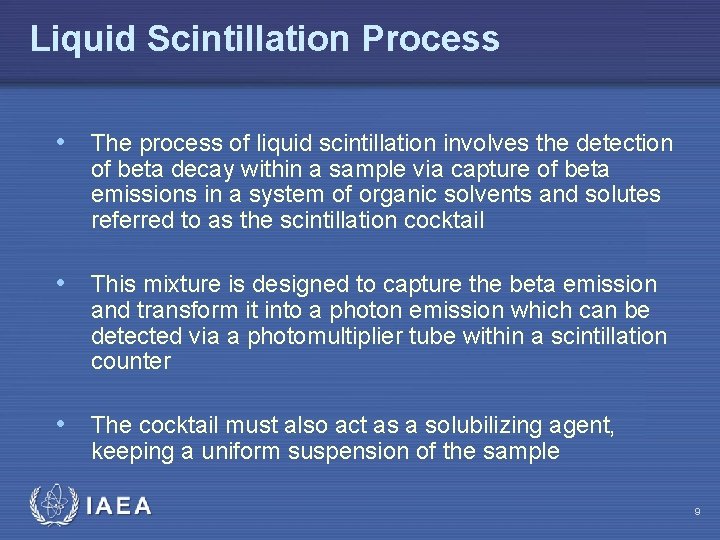
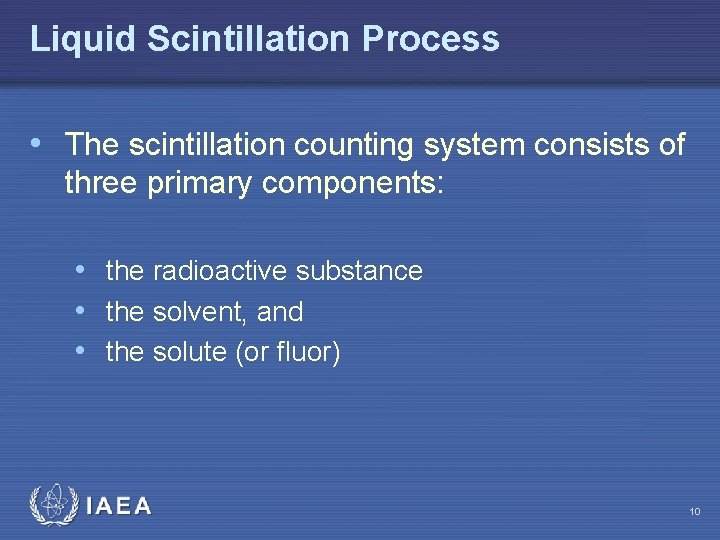
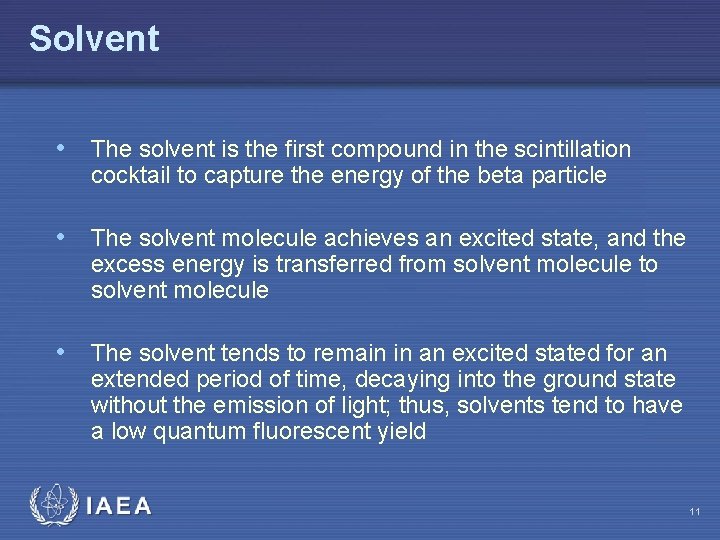
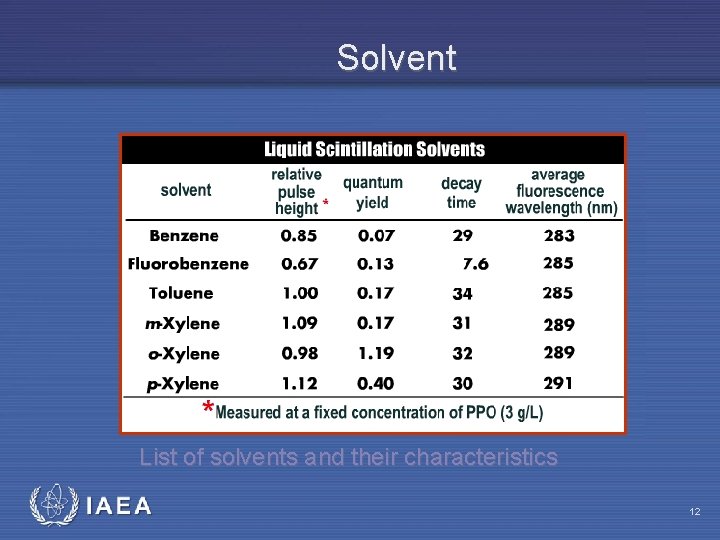
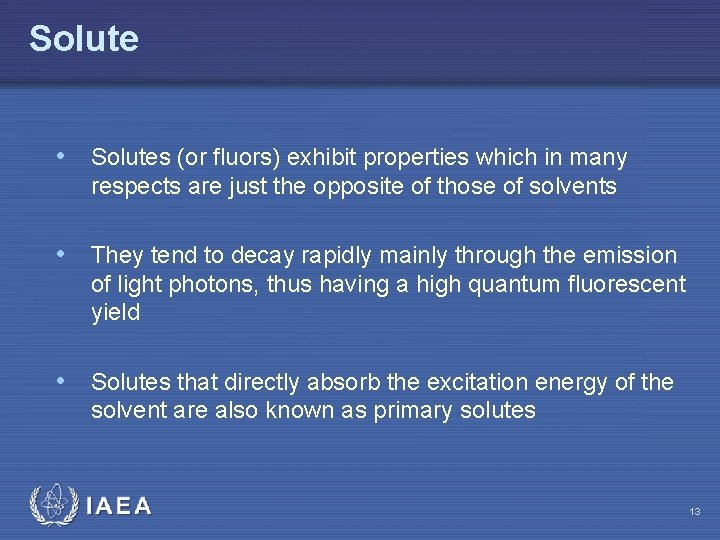
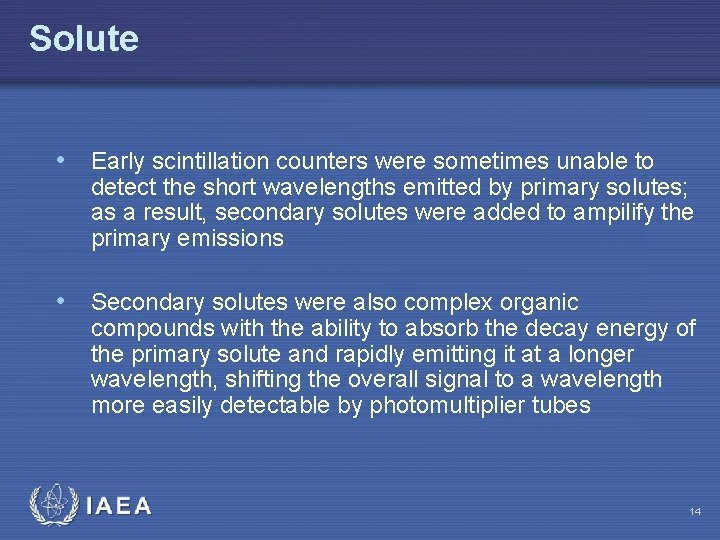
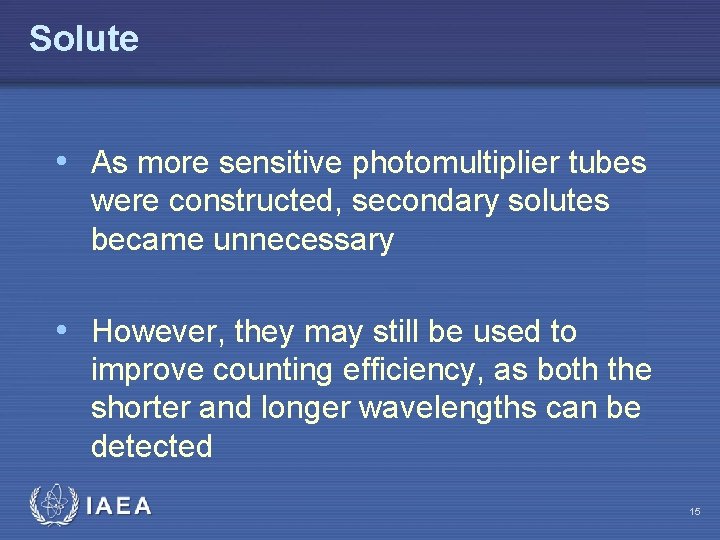
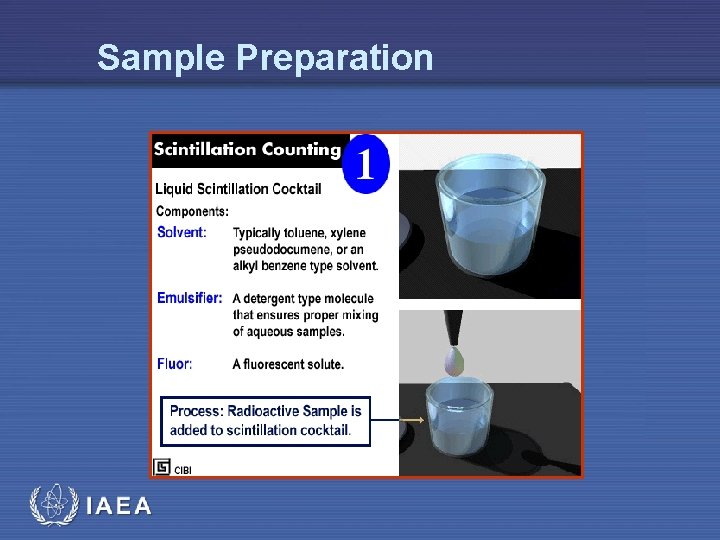
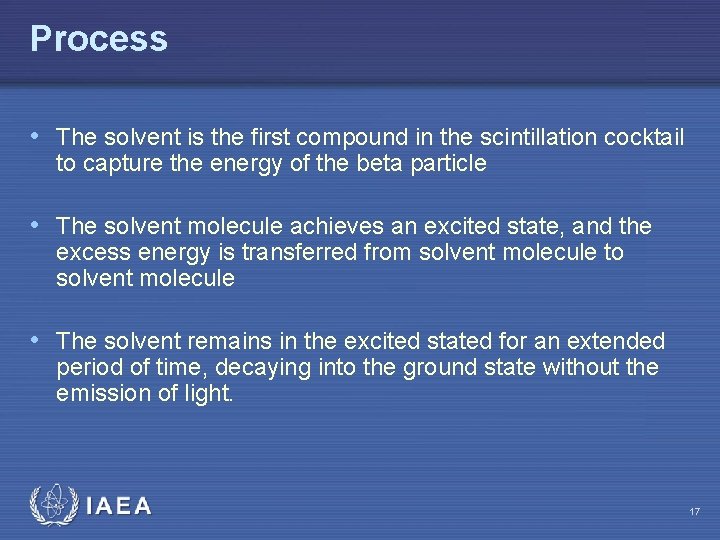
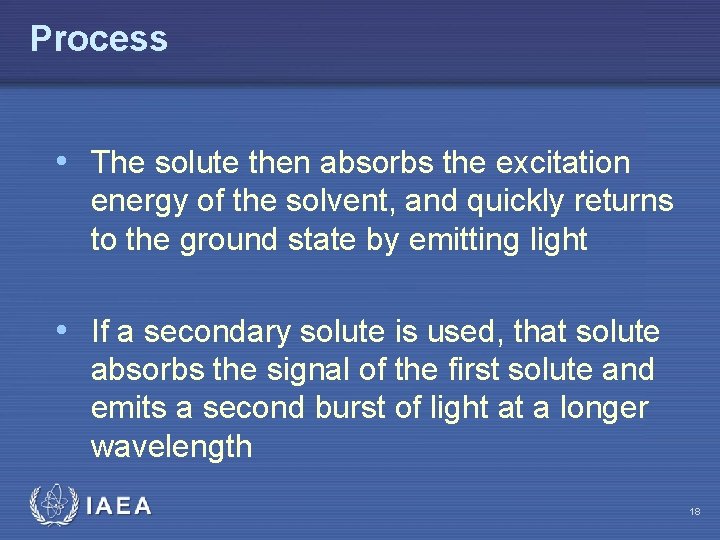
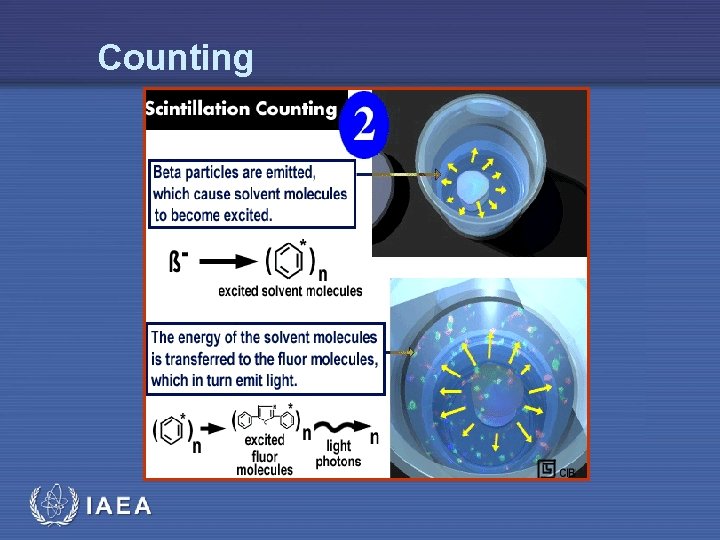
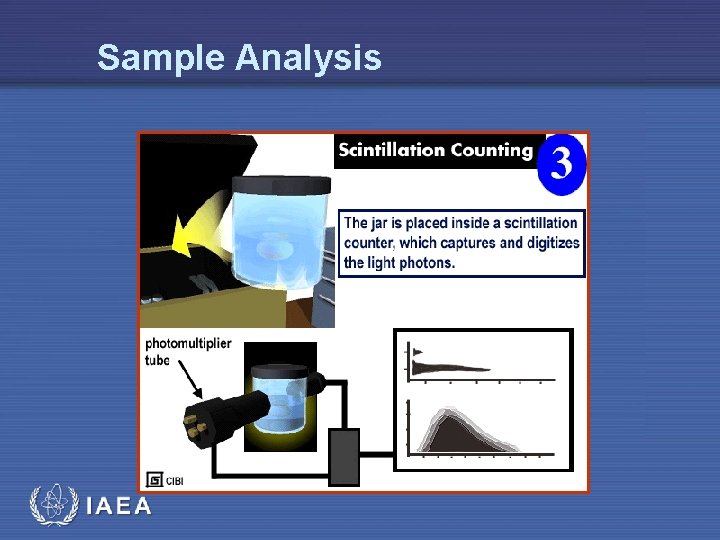
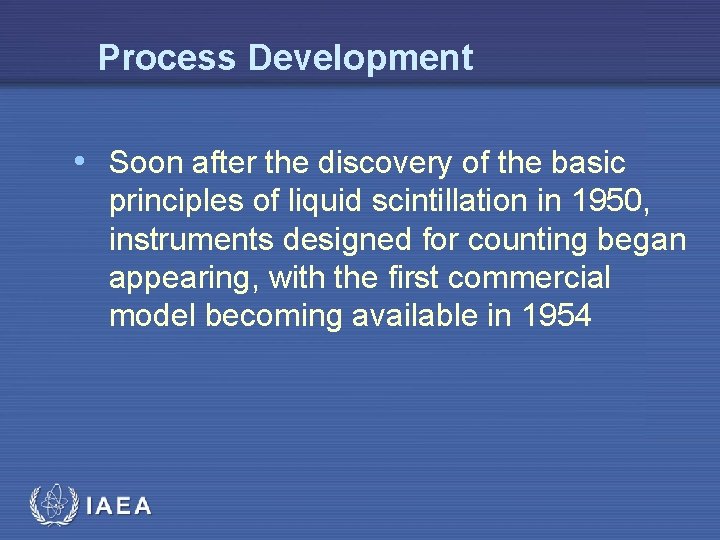
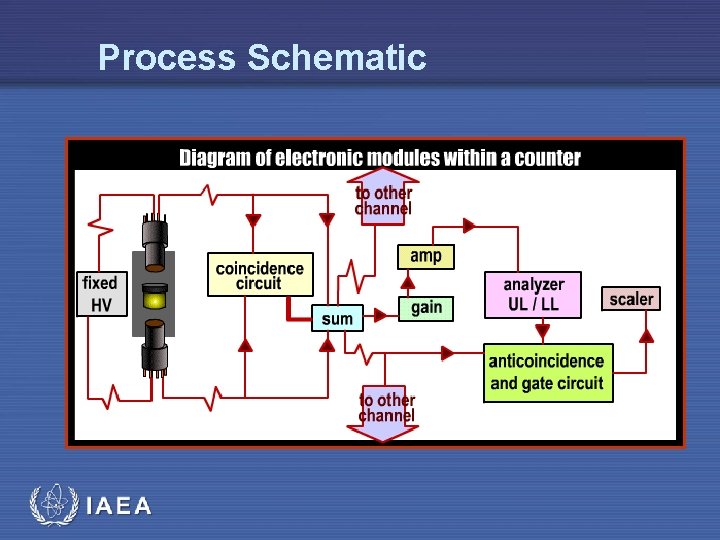
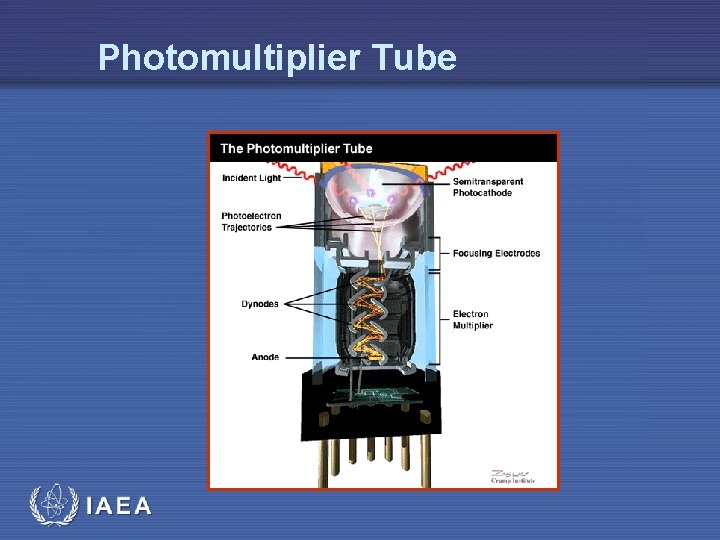
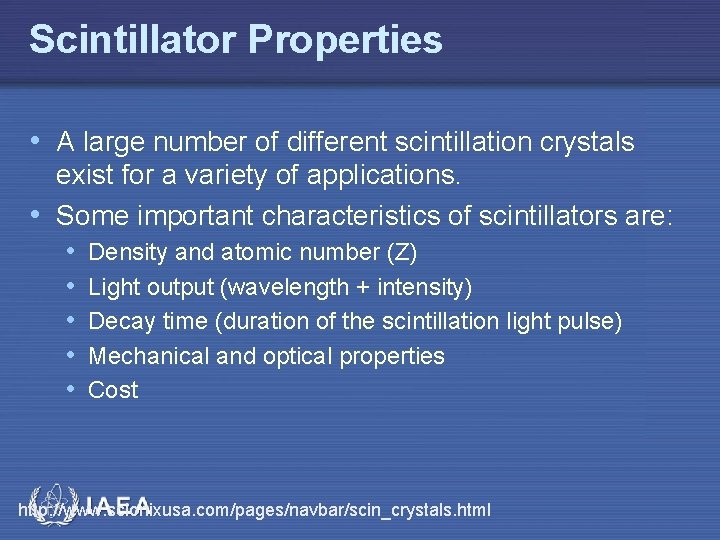
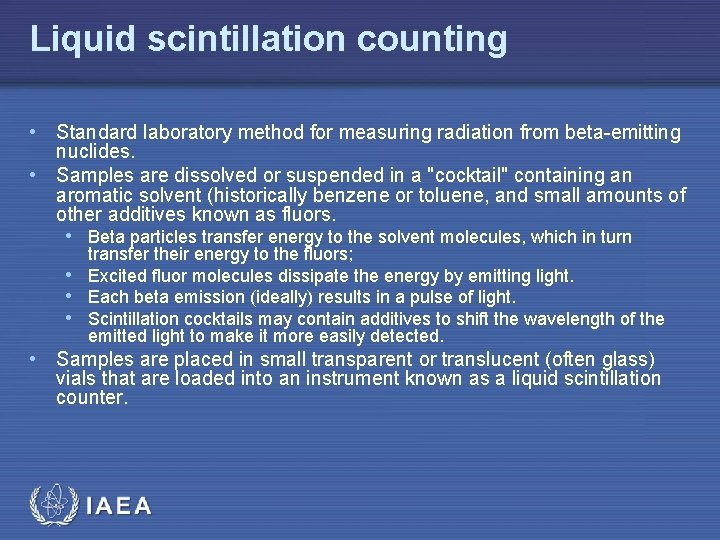
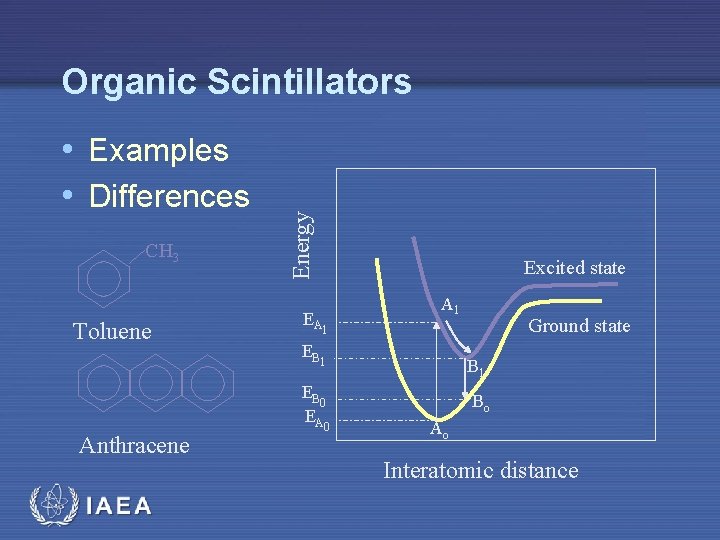
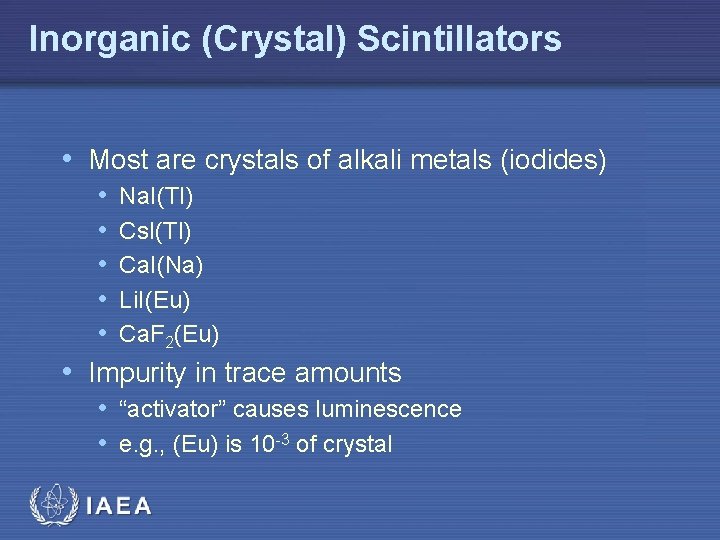
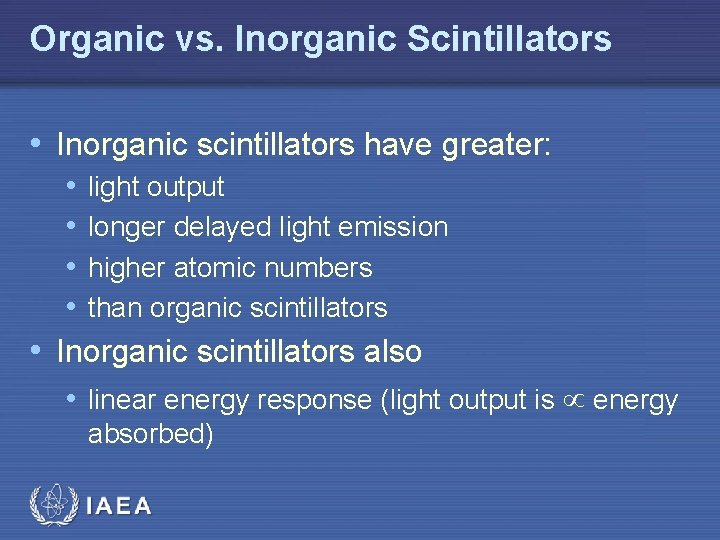
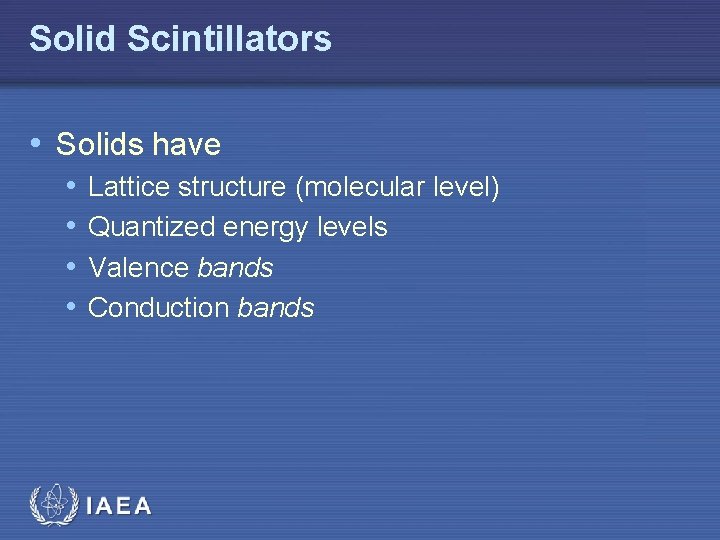
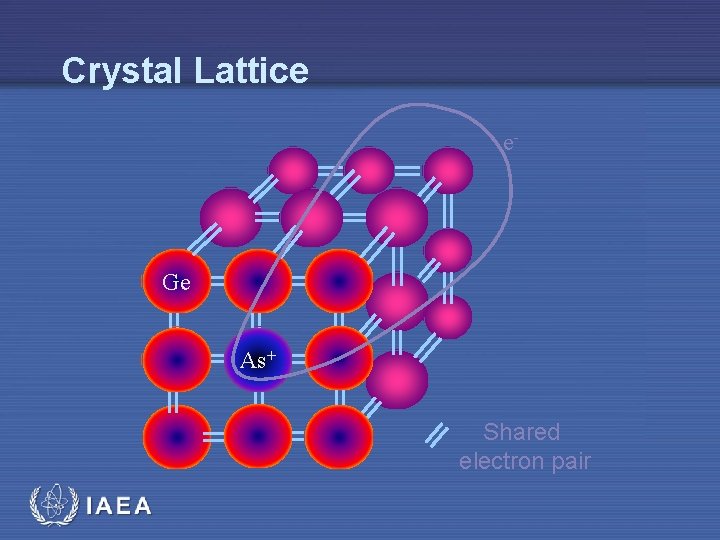
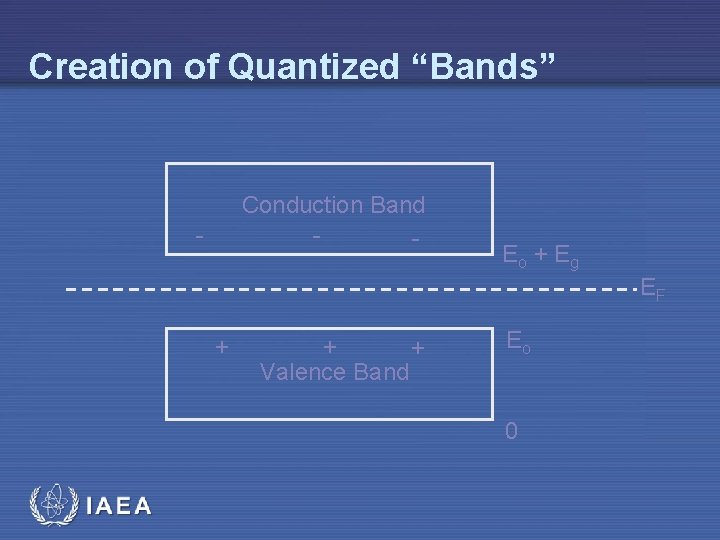
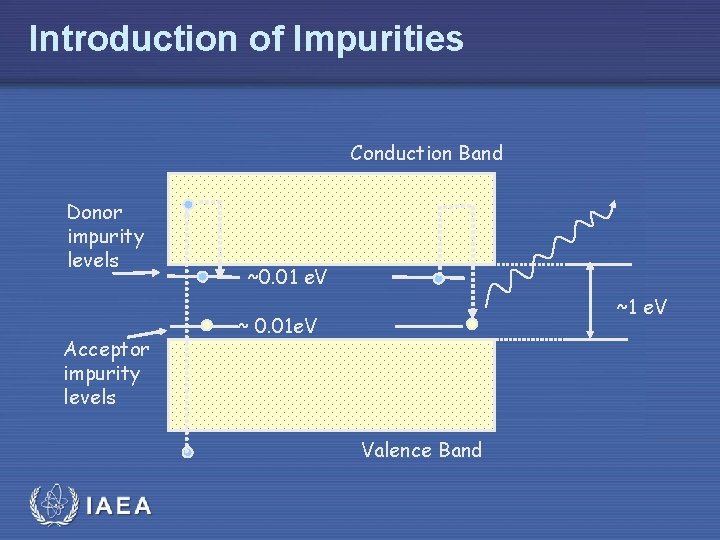
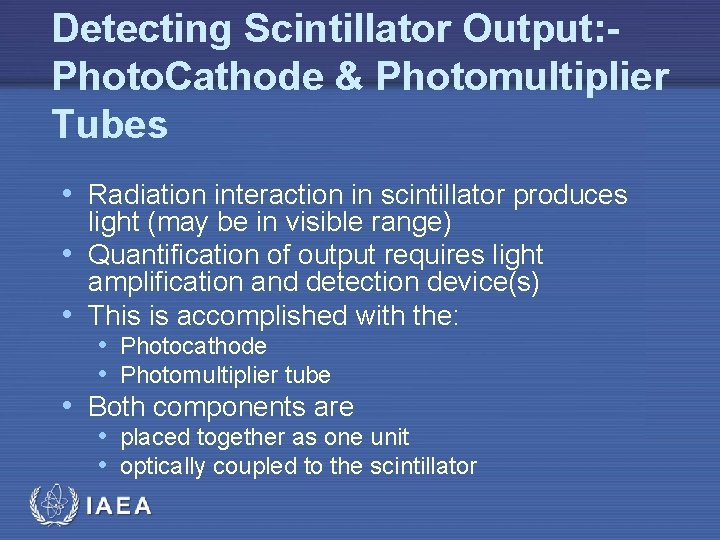
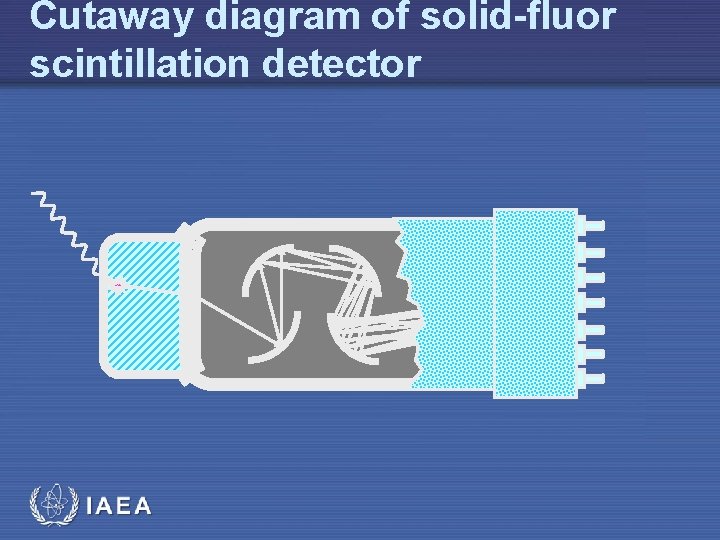
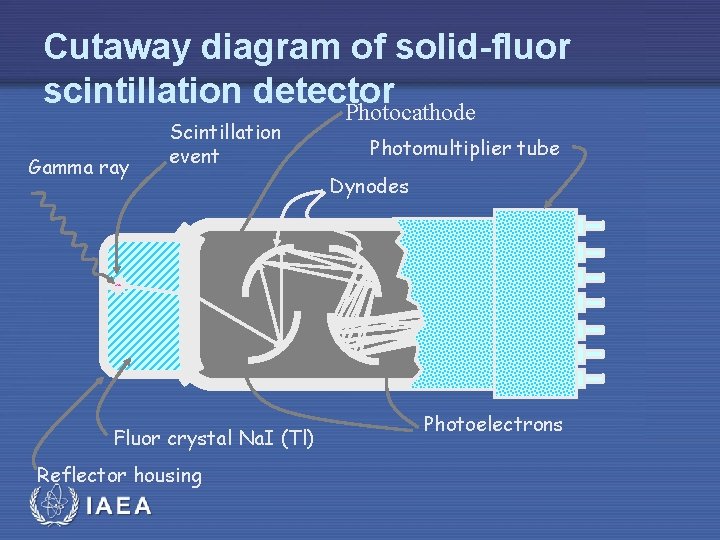
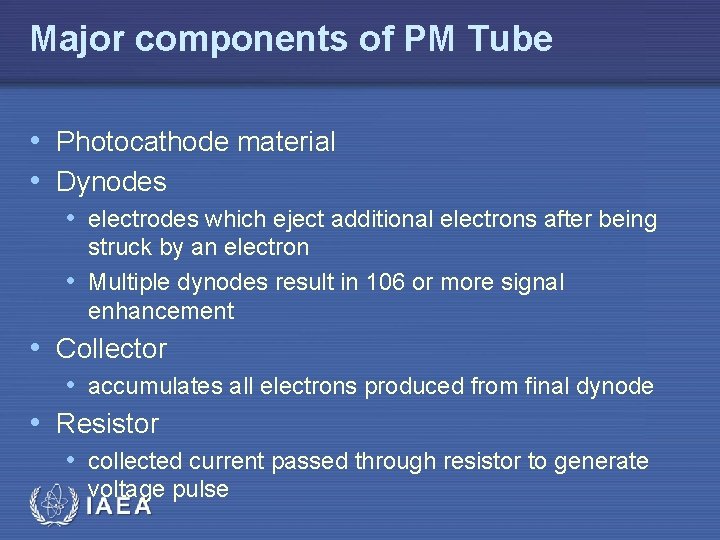
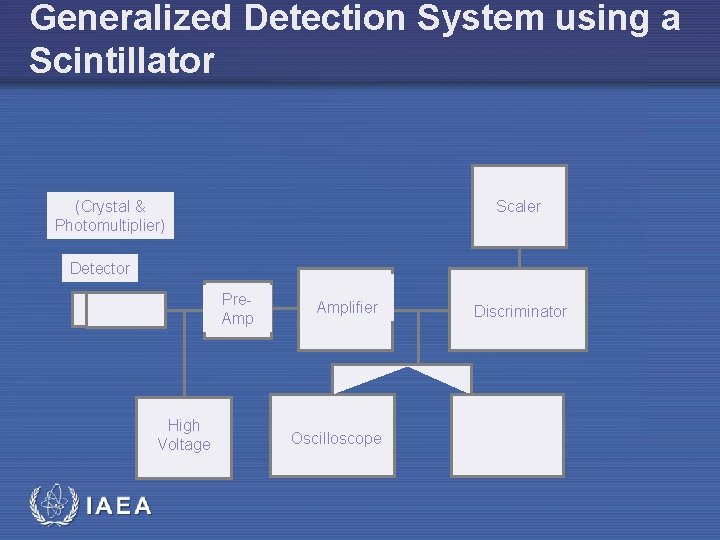
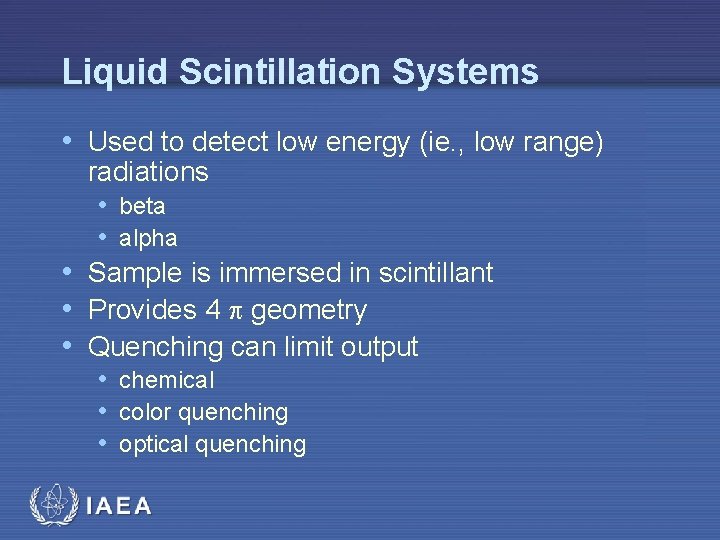
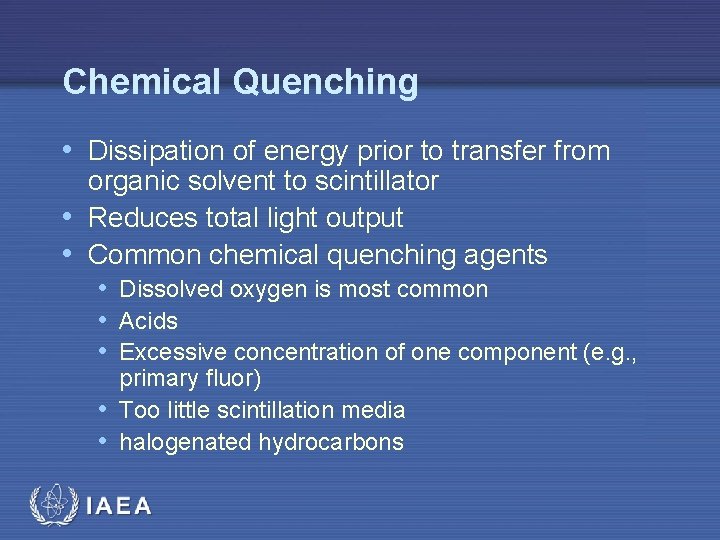
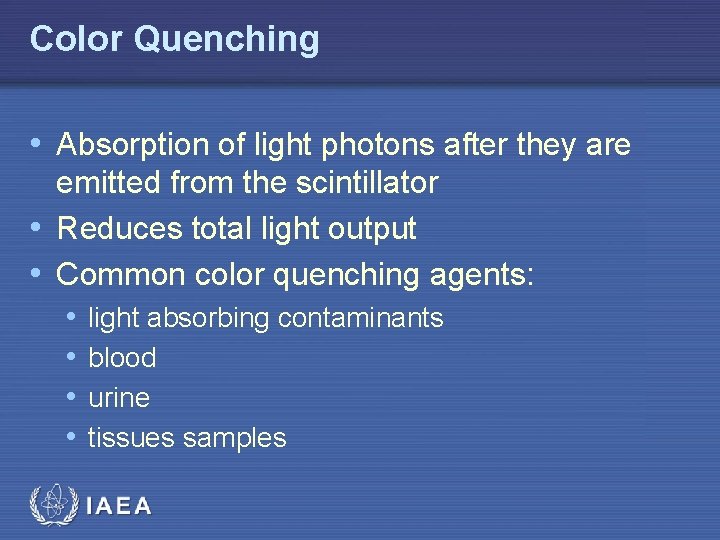
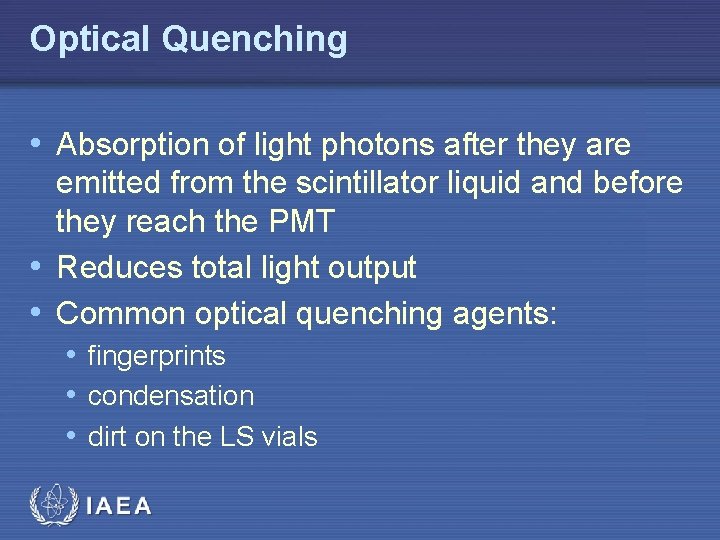
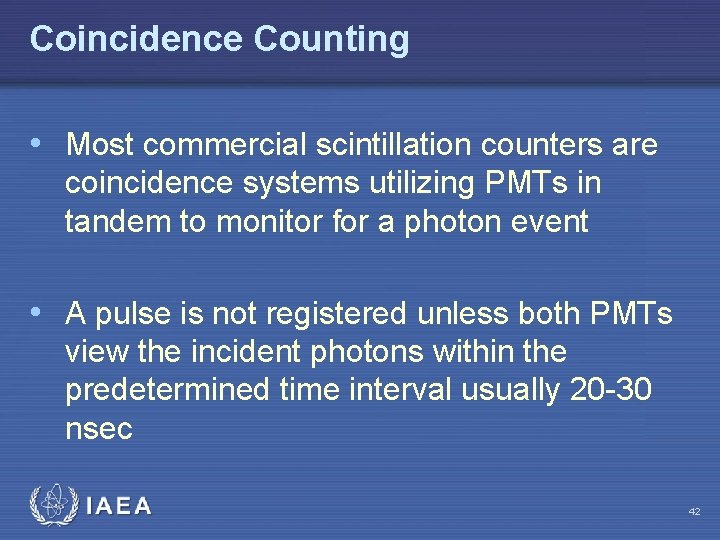
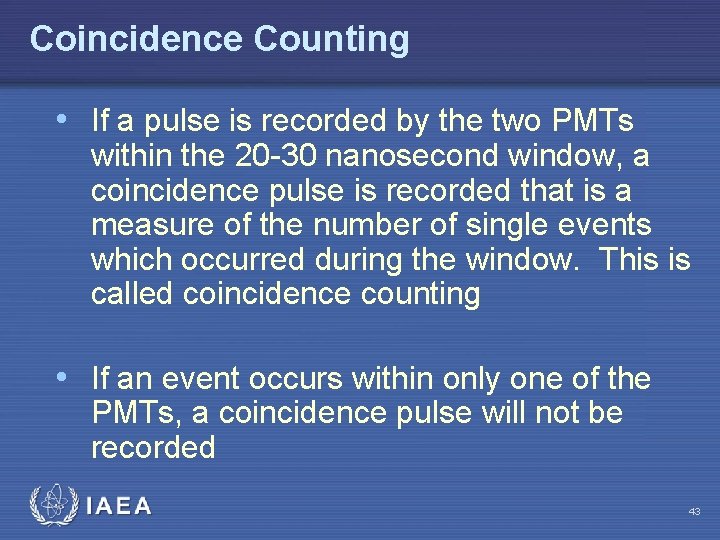
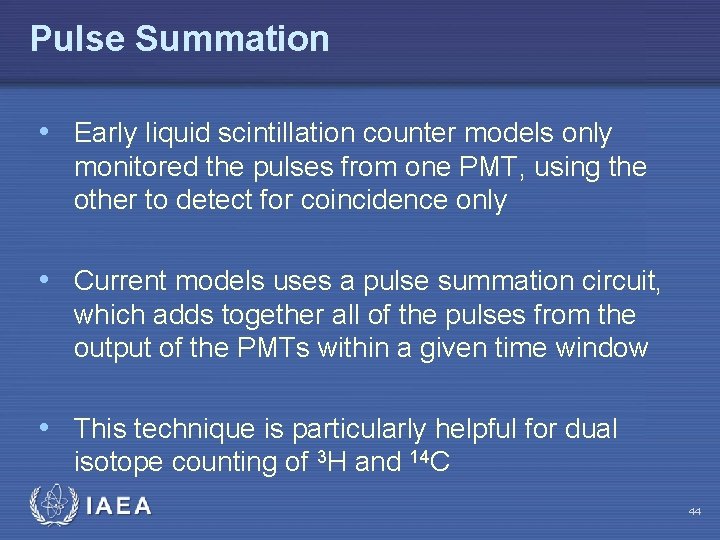
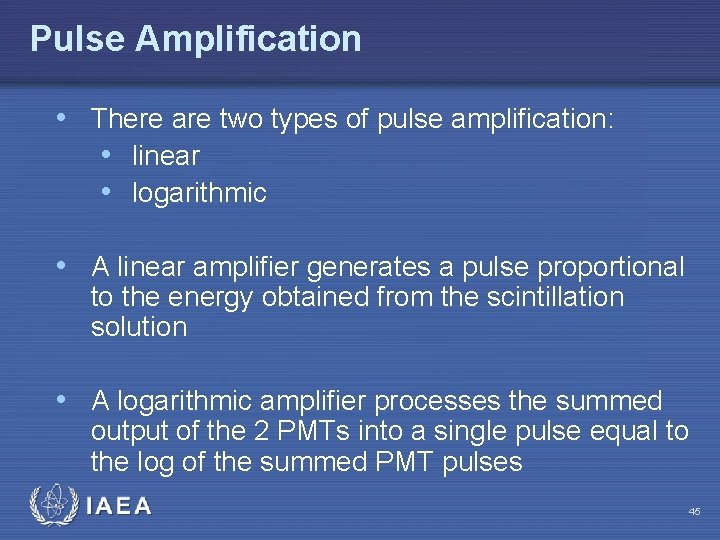
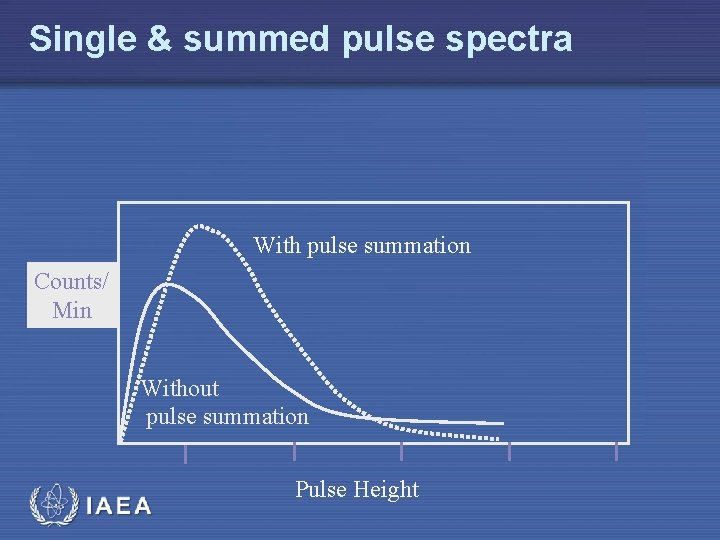
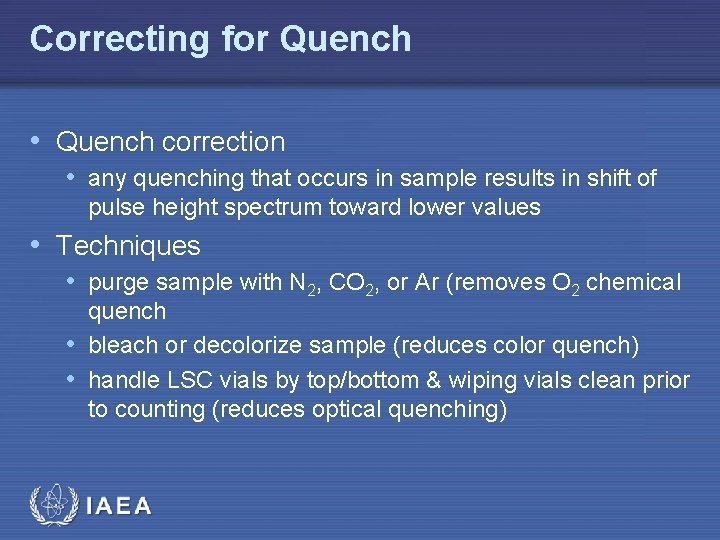
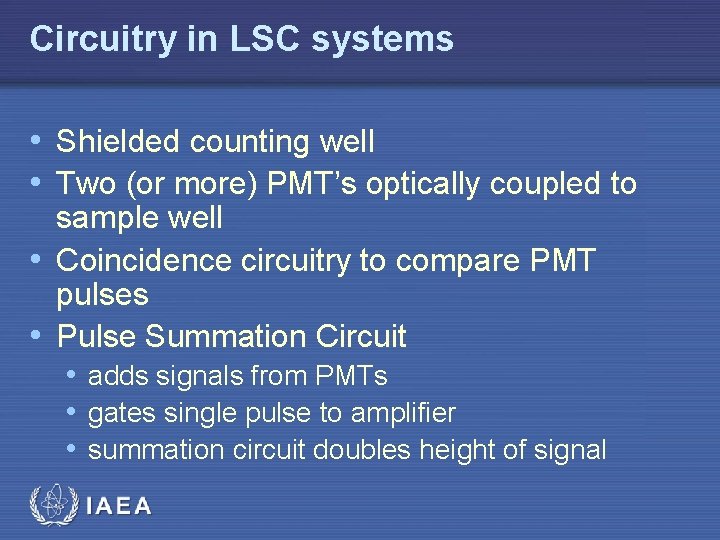
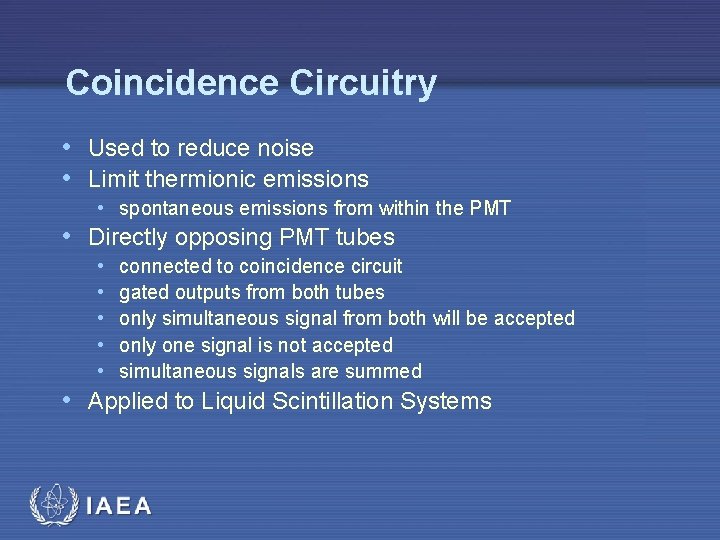
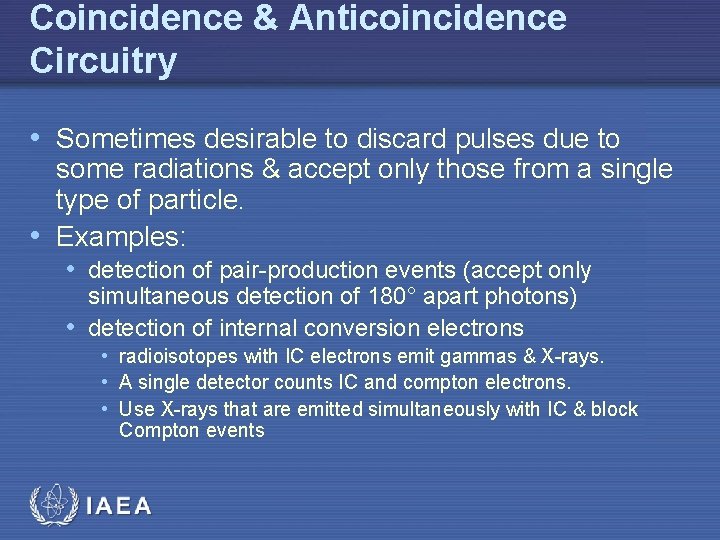
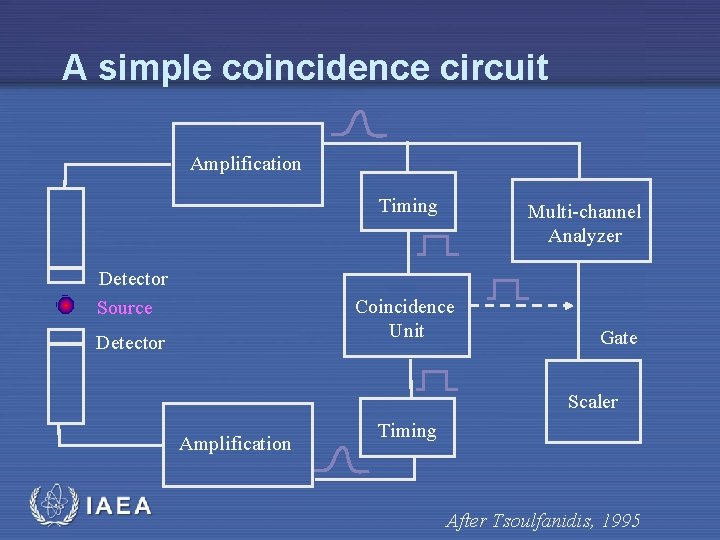
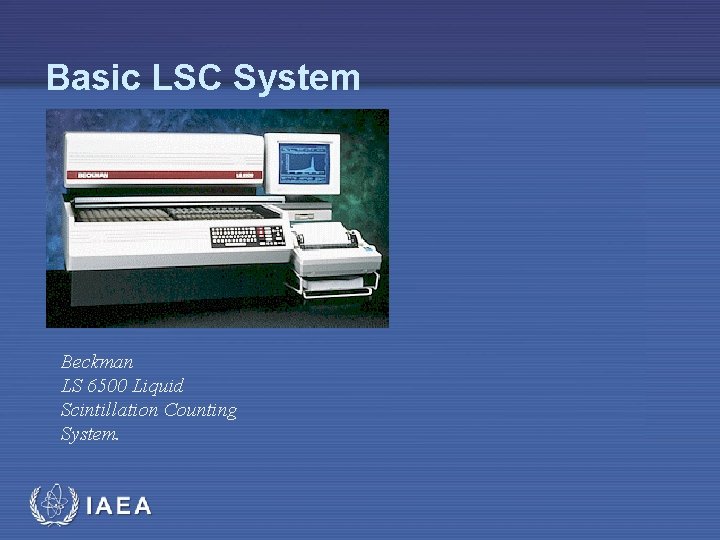
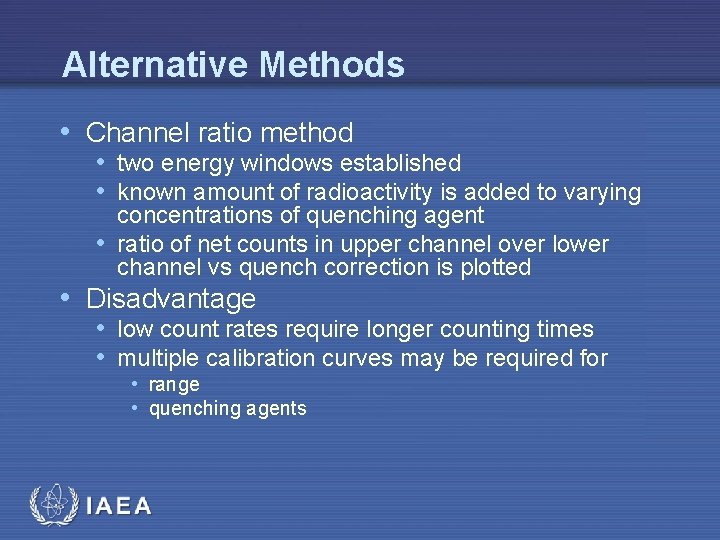
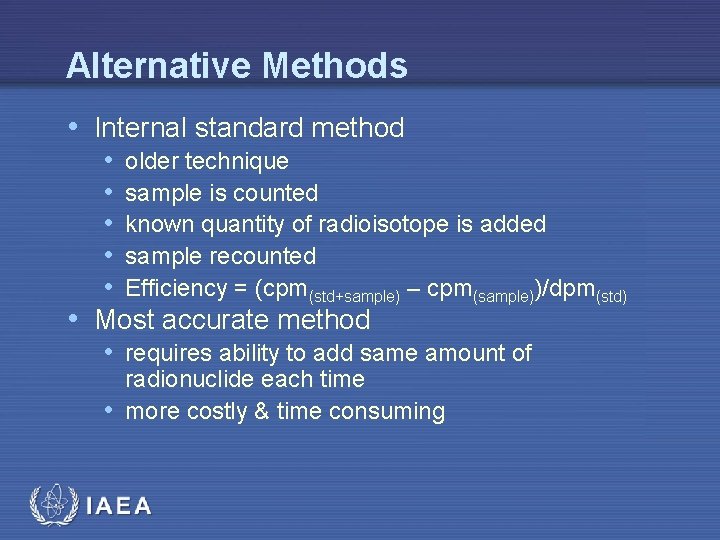
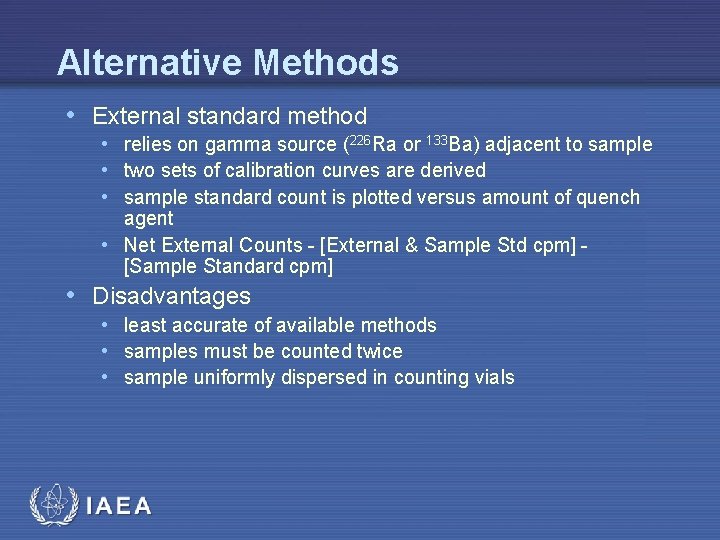
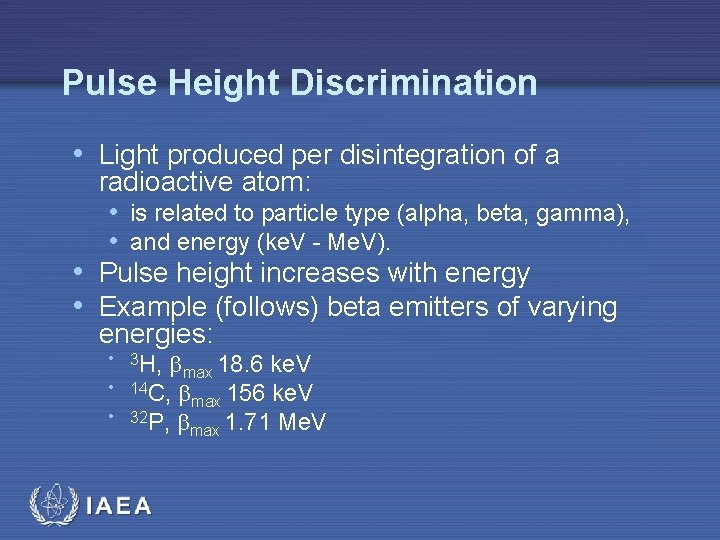
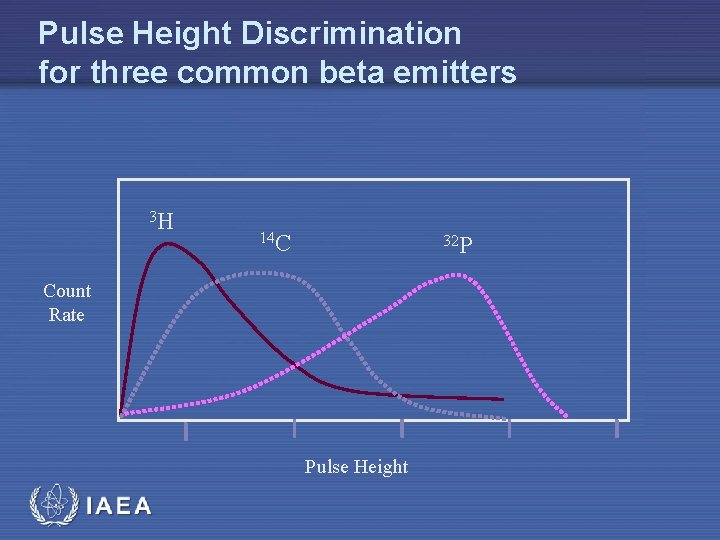
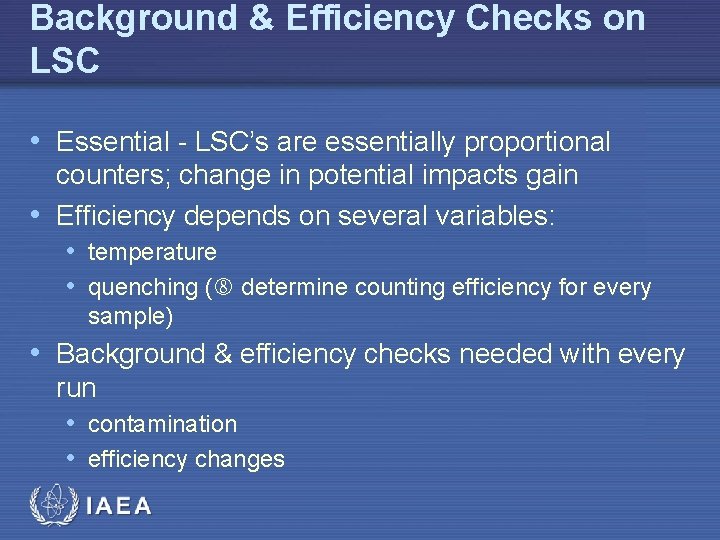
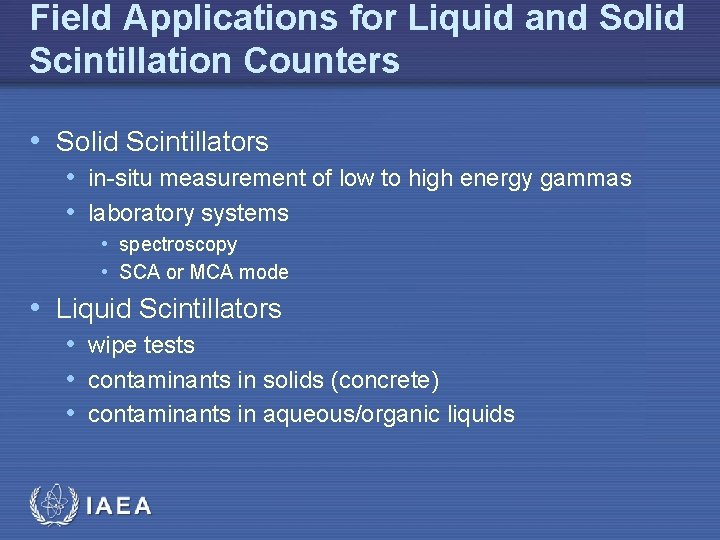
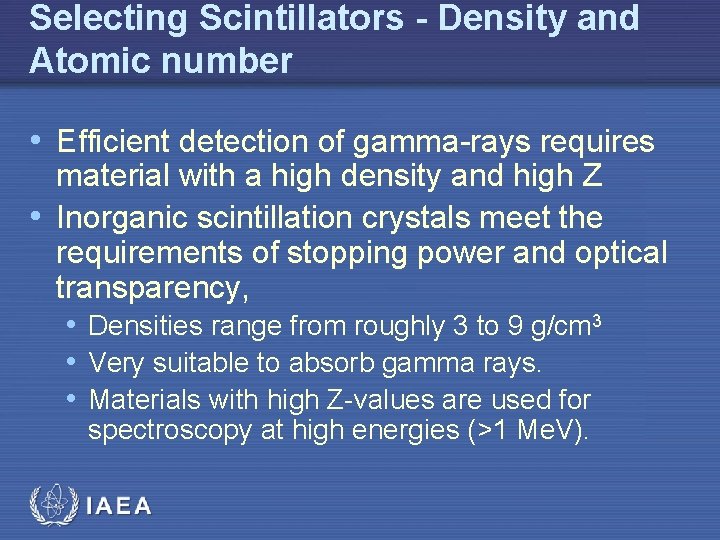
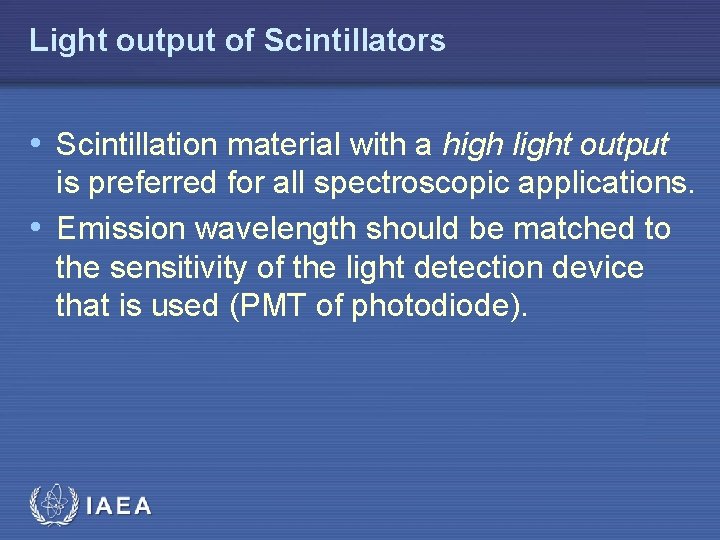
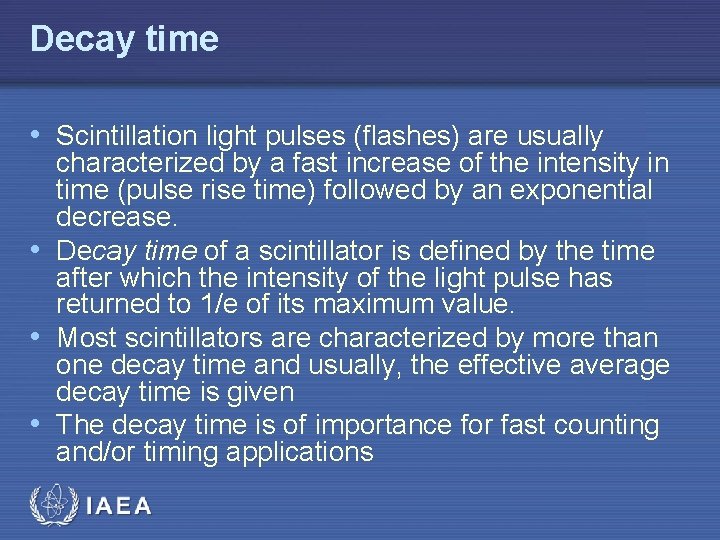
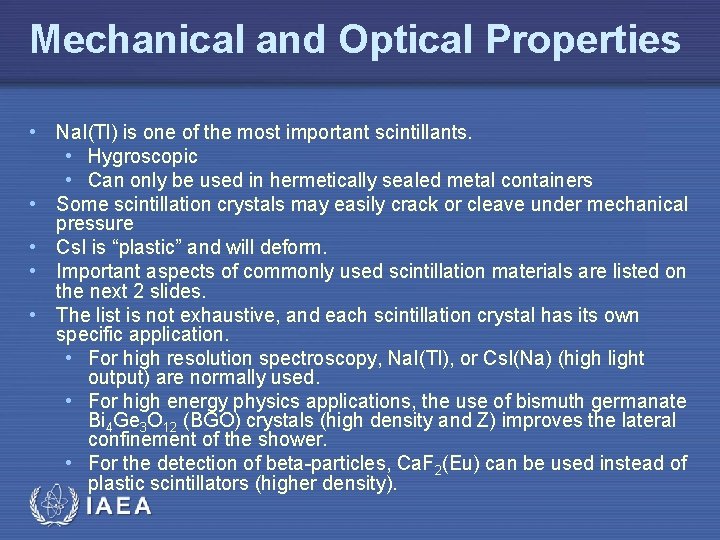
![Commonly Used Scintillators Density [g/cm 3] Emission Max [nm] Decay Constant (1) Refractive Index Commonly Used Scintillators Density [g/cm 3] Emission Max [nm] Decay Constant (1) Refractive Index](https://slidetodoc.com/presentation_image/7e3d3ae3479150717d2fd9fb070c1b0f/image-64.jpg)
![Commonly Used Scintillators Material Density [g/cm 3] Emission Maximum [nm] Decay Constant (1) Refractive Commonly Used Scintillators Material Density [g/cm 3] Emission Maximum [nm] Decay Constant (1) Refractive](https://slidetodoc.com/presentation_image/7e3d3ae3479150717d2fd9fb070c1b0f/image-65.jpg)
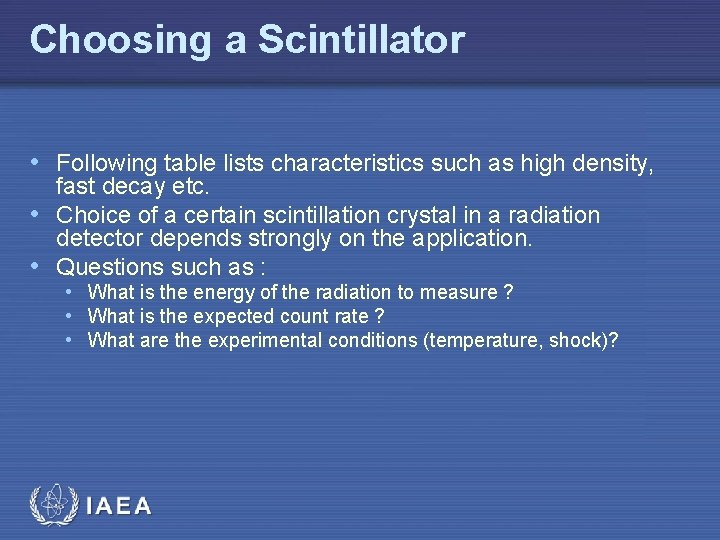
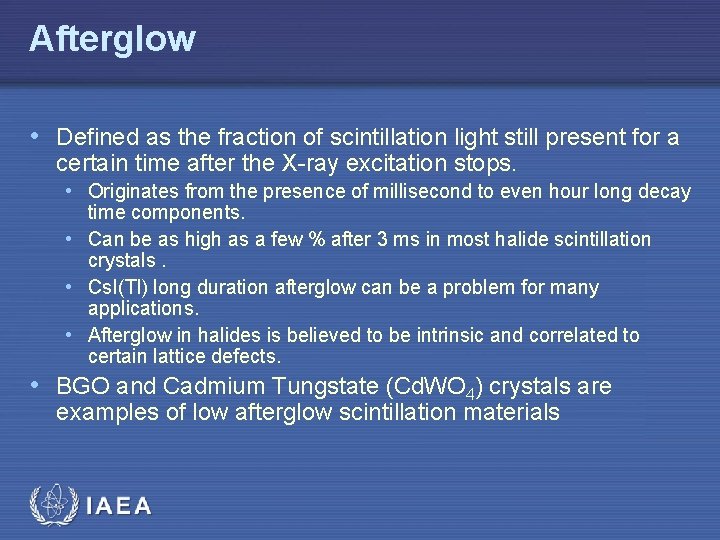
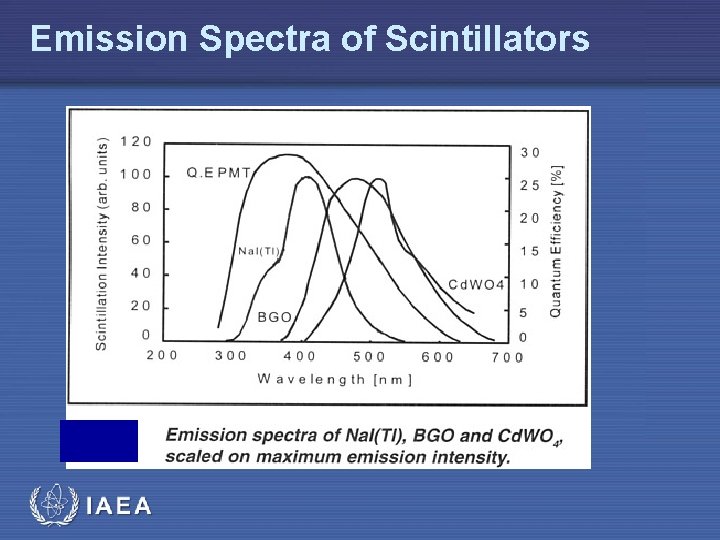
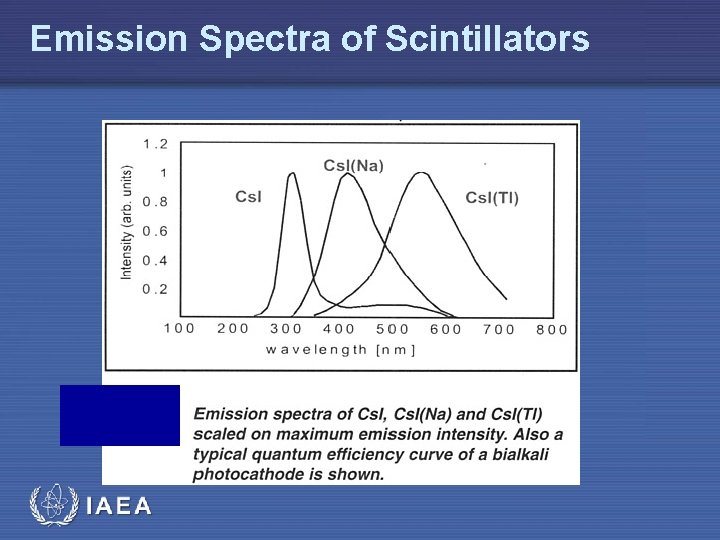
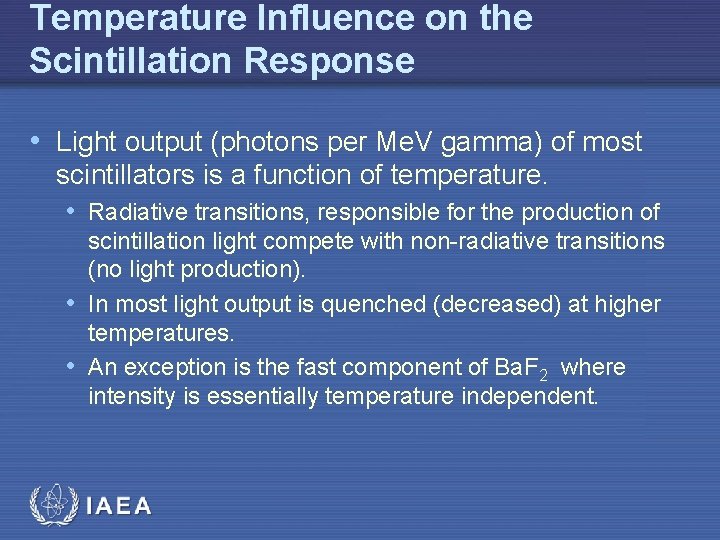
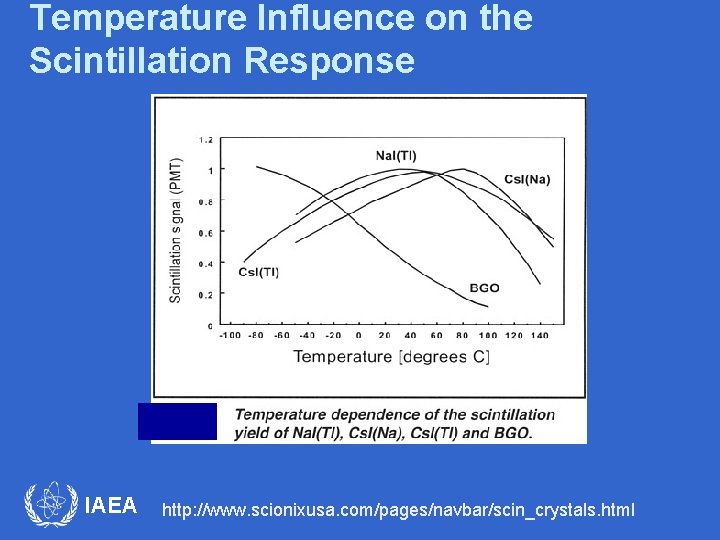
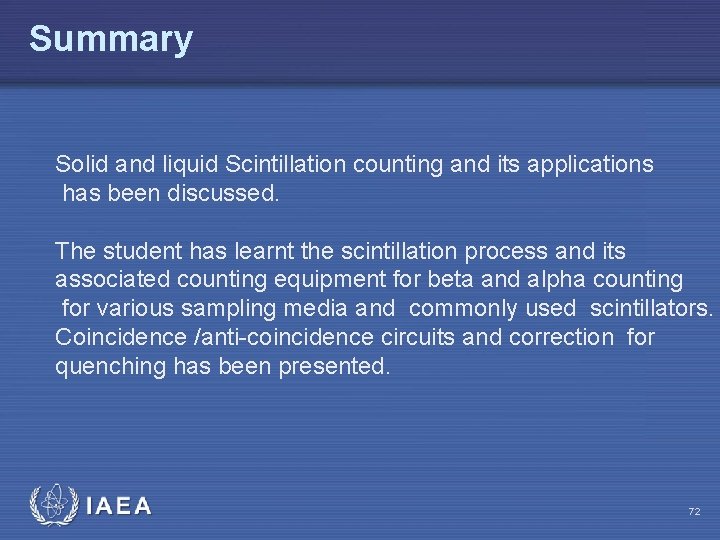
- Slides: 72
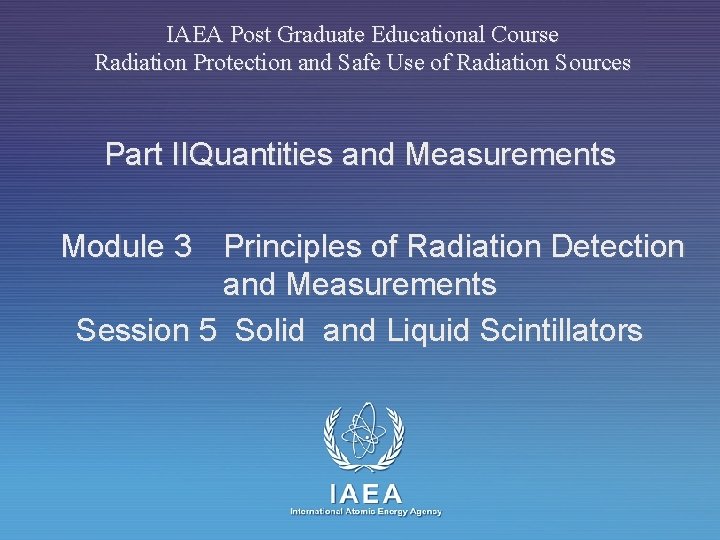
IAEA Post Graduate Educational Course Radiation Protection and Safe Use of Radiation Sources Part IIQuantities and Measurements Module 3 Principles of Radiation Detection and Measurements Session 5 Solid and Liquid Scintillators IAEA International Atomic Energy Agency
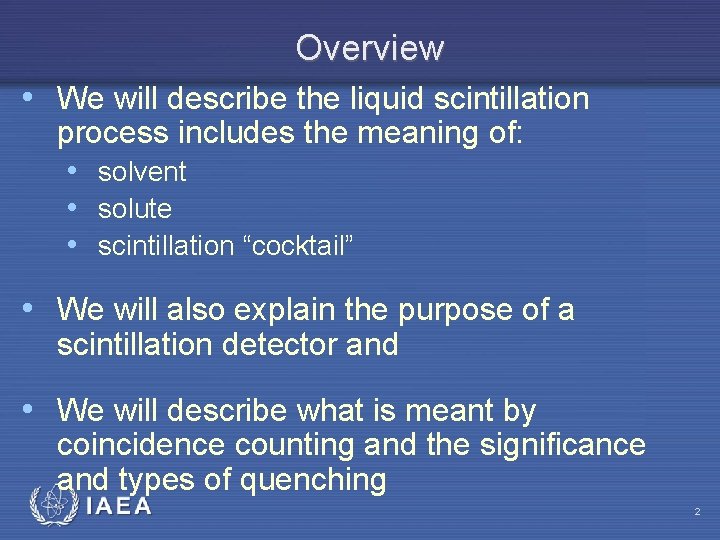
Overview • We will describe the liquid scintillation process includes the meaning of: • solvent • solute • scintillation “cocktail” • We will also explain the purpose of a scintillation detector and • We will describe what is meant by coincidence counting and the significance and types of quenching IAEA 2
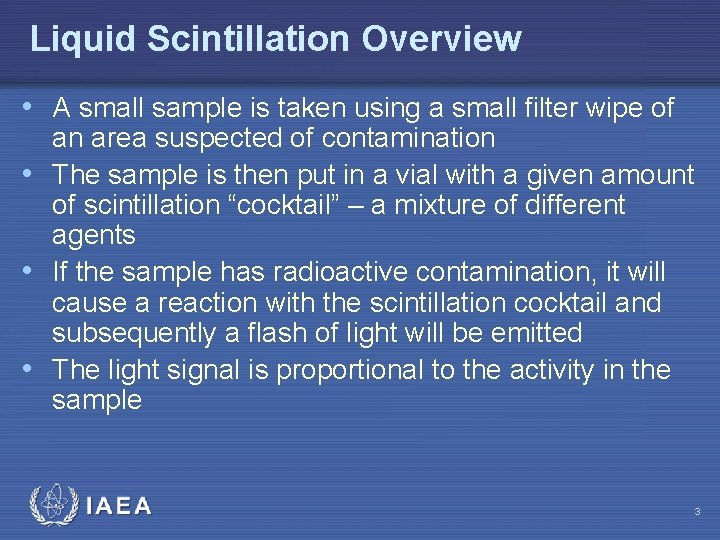
Liquid Scintillation Overview • A small sample is taken using a small filter wipe of an area suspected of contamination • The sample is then put in a vial with a given amount of scintillation “cocktail” – a mixture of different agents • If the sample has radioactive contamination, it will cause a reaction with the scintillation cocktail and subsequently a flash of light will be emitted • The light signal is proportional to the activity in the sample IAEA 3
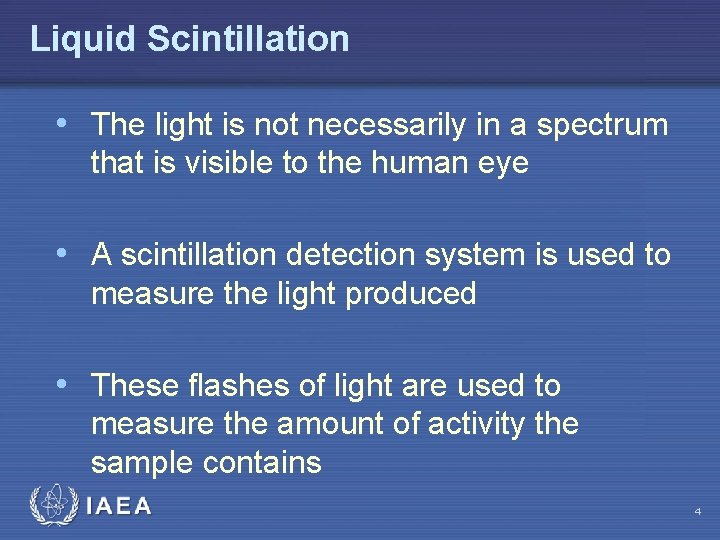
Liquid Scintillation • The light is not necessarily in a spectrum that is visible to the human eye • A scintillation detection system is used to measure the light produced • These flashes of light are used to measure the amount of activity the sample contains IAEA 4
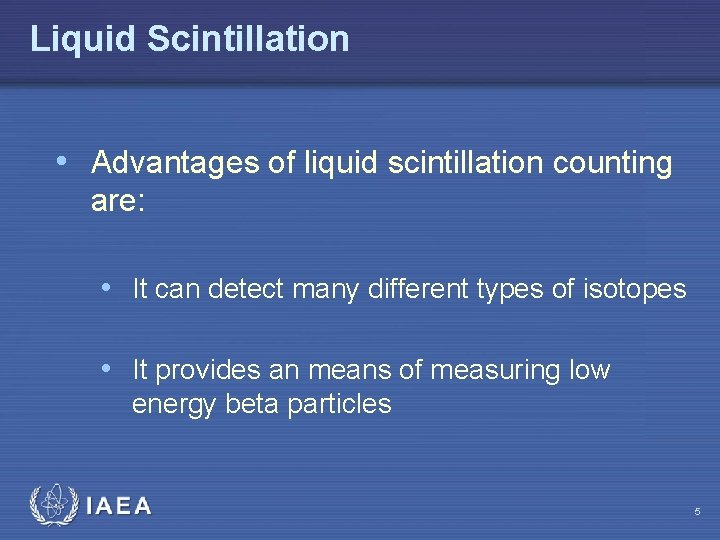
Liquid Scintillation • Advantages of liquid scintillation counting are: • It can detect many different types of isotopes • It provides an means of measuring low energy beta particles IAEA 5
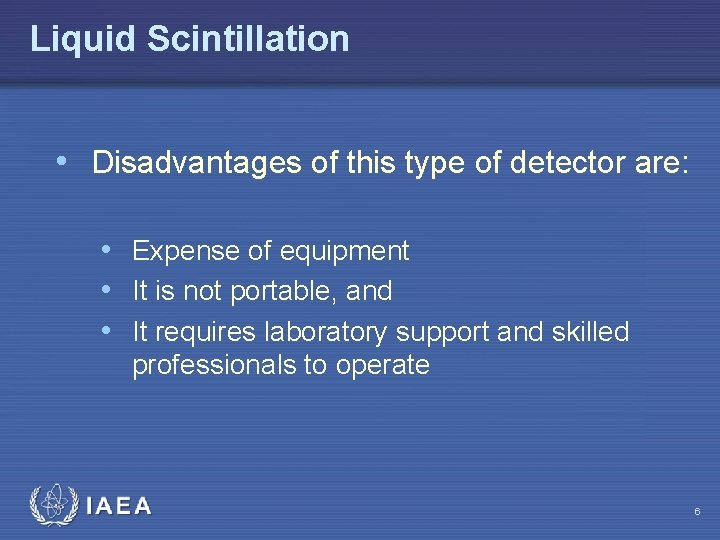
Liquid Scintillation • Disadvantages of this type of detector are: • Expense of equipment • It is not portable, and • It requires laboratory support and skilled professionals to operate IAEA 6
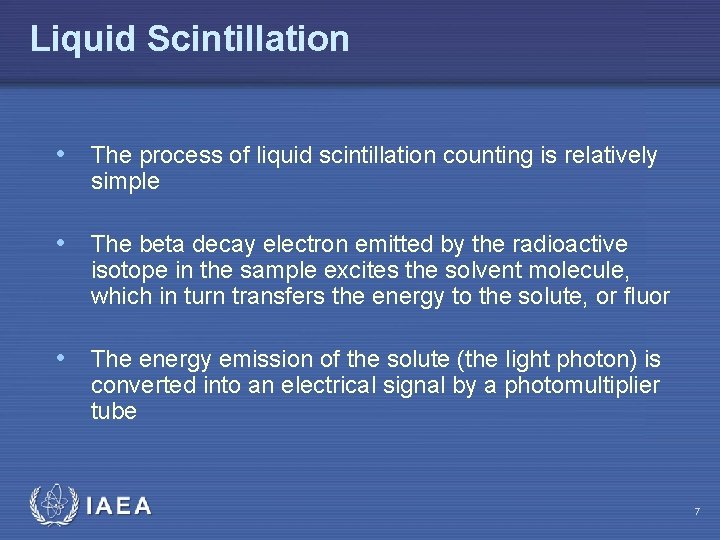
Liquid Scintillation • The process of liquid scintillation counting is relatively simple • The beta decay electron emitted by the radioactive isotope in the sample excites the solvent molecule, which in turn transfers the energy to the solute, or fluor • The energy emission of the solute (the light photon) is converted into an electrical signal by a photomultiplier tube IAEA 7
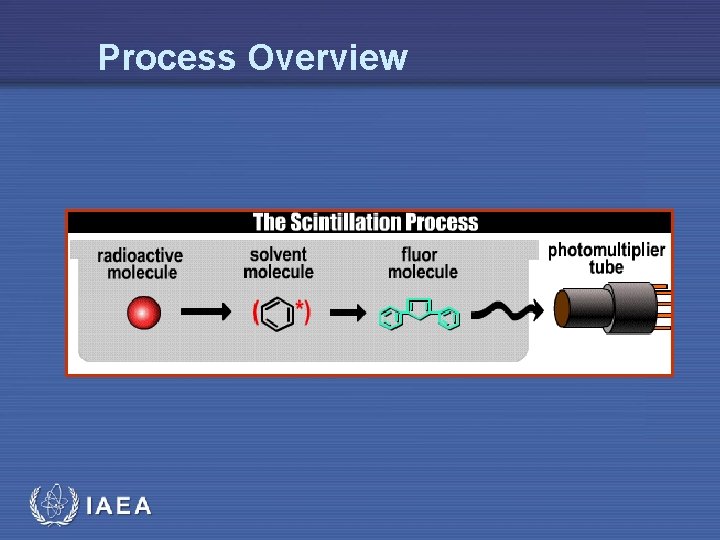
Process Overview IAEA
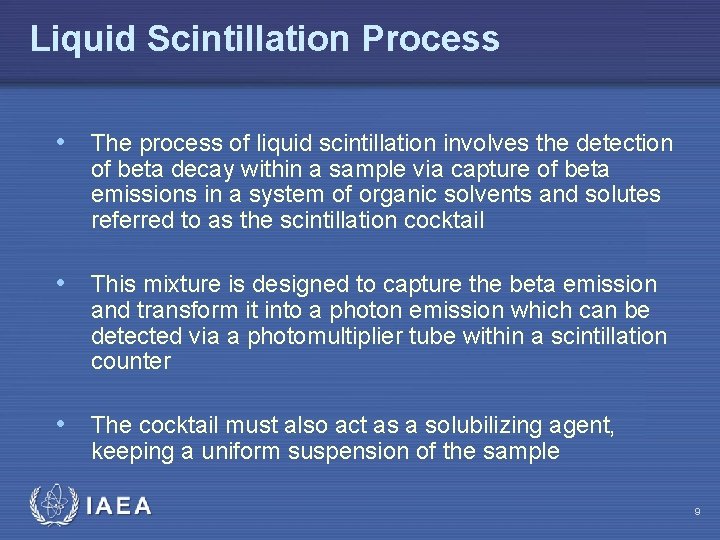
Liquid Scintillation Process • The process of liquid scintillation involves the detection of beta decay within a sample via capture of beta emissions in a system of organic solvents and solutes referred to as the scintillation cocktail • This mixture is designed to capture the beta emission and transform it into a photon emission which can be detected via a photomultiplier tube within a scintillation counter • The cocktail must also act as a solubilizing agent, keeping a uniform suspension of the sample IAEA 9
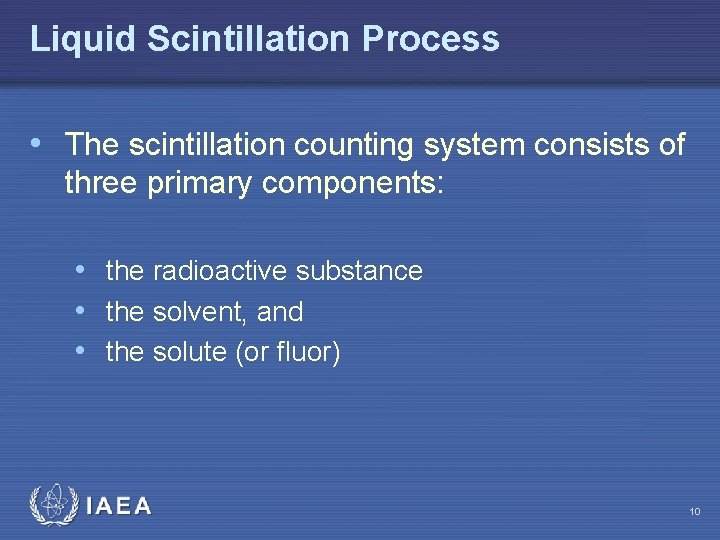
Liquid Scintillation Process • The scintillation counting system consists of three primary components: • the radioactive substance • the solvent, and • the solute (or fluor) IAEA 10
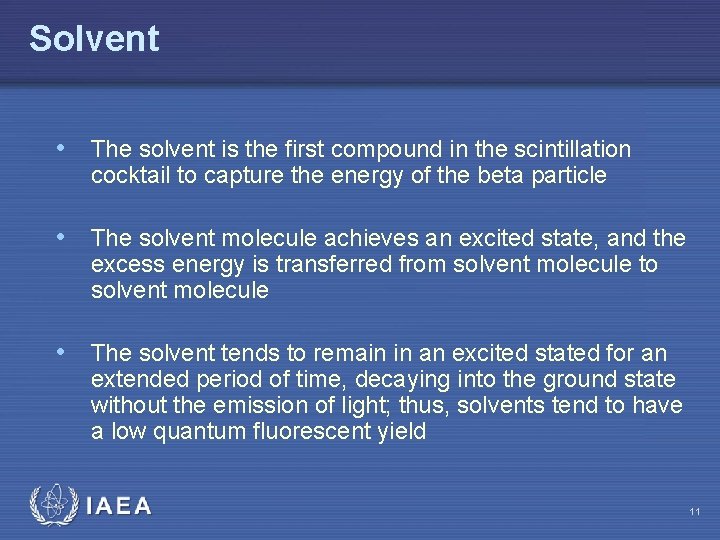
Solvent • The solvent is the first compound in the scintillation cocktail to capture the energy of the beta particle • The solvent molecule achieves an excited state, and the excess energy is transferred from solvent molecule to solvent molecule • The solvent tends to remain in an excited stated for an extended period of time, decaying into the ground state without the emission of light; thus, solvents tend to have a low quantum fluorescent yield IAEA 11
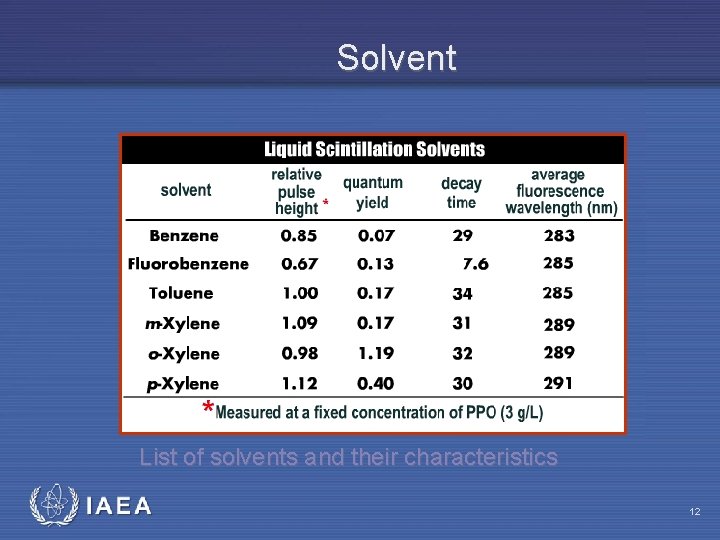
Solvent List of solvents and their characteristics IAEA 12
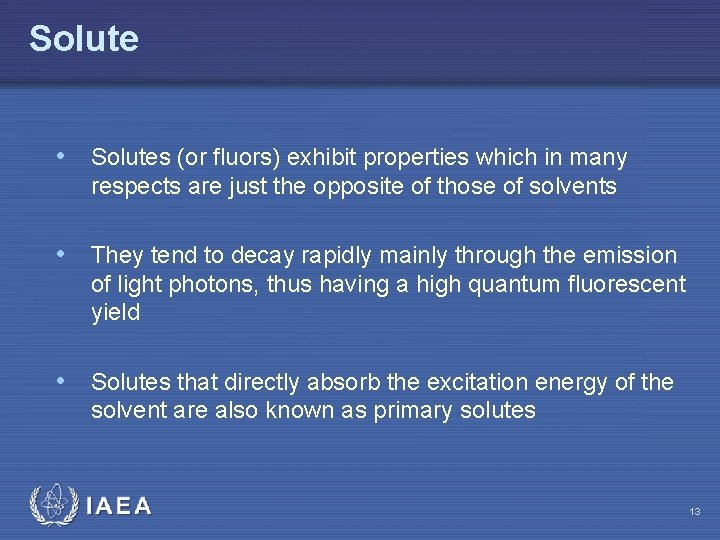
Solute • Solutes (or fluors) exhibit properties which in many respects are just the opposite of those of solvents • They tend to decay rapidly mainly through the emission of light photons, thus having a high quantum fluorescent yield • Solutes that directly absorb the excitation energy of the solvent are also known as primary solutes IAEA 13
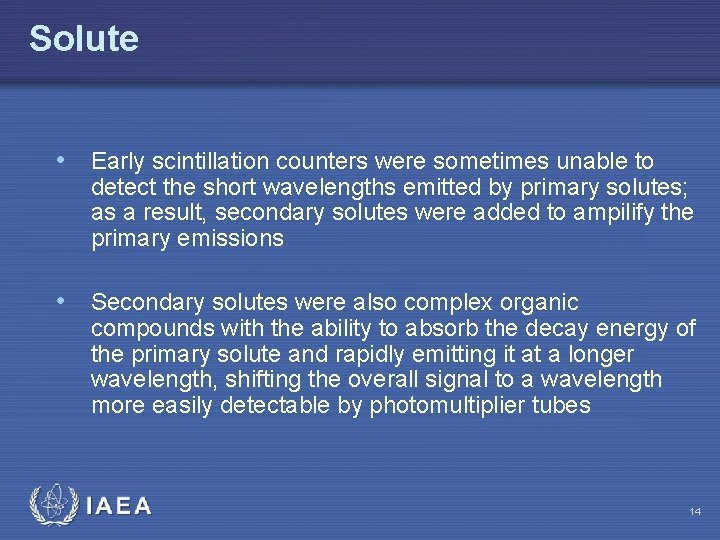
Solute • Early scintillation counters were sometimes unable to detect the short wavelengths emitted by primary solutes; as a result, secondary solutes were added to ampilify the primary emissions • Secondary solutes were also complex organic compounds with the ability to absorb the decay energy of the primary solute and rapidly emitting it at a longer wavelength, shifting the overall signal to a wavelength more easily detectable by photomultiplier tubes IAEA 14
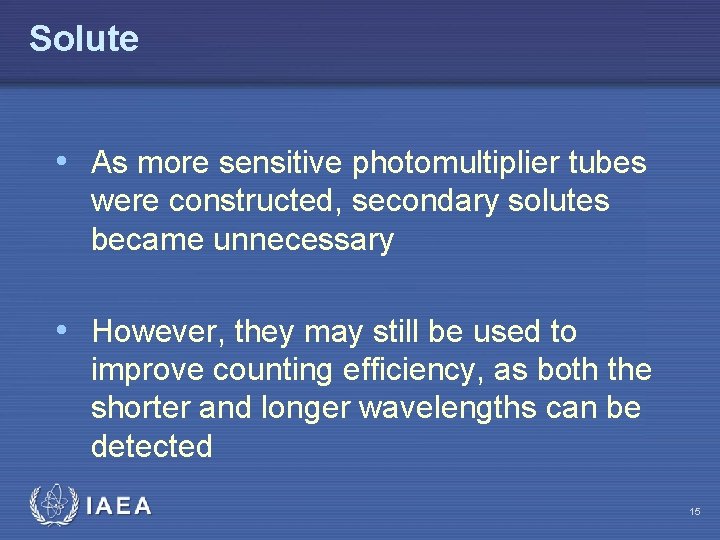
Solute • As more sensitive photomultiplier tubes were constructed, secondary solutes became unnecessary • However, they may still be used to improve counting efficiency, as both the shorter and longer wavelengths can be detected IAEA 15
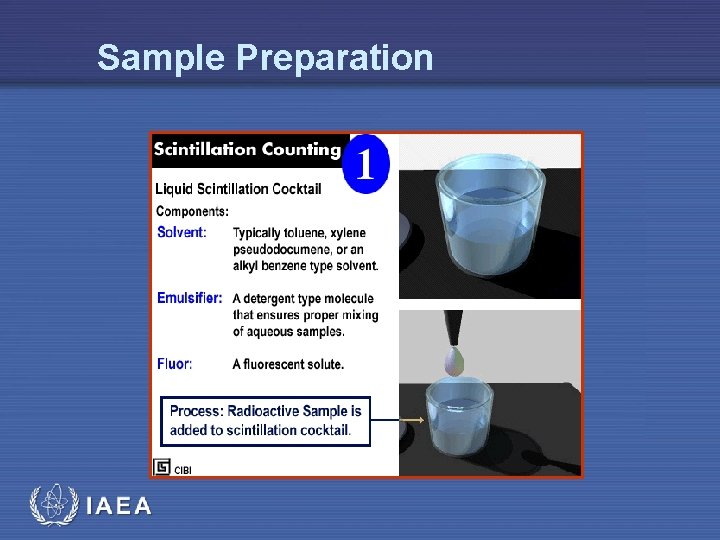
Sample Preparation IAEA
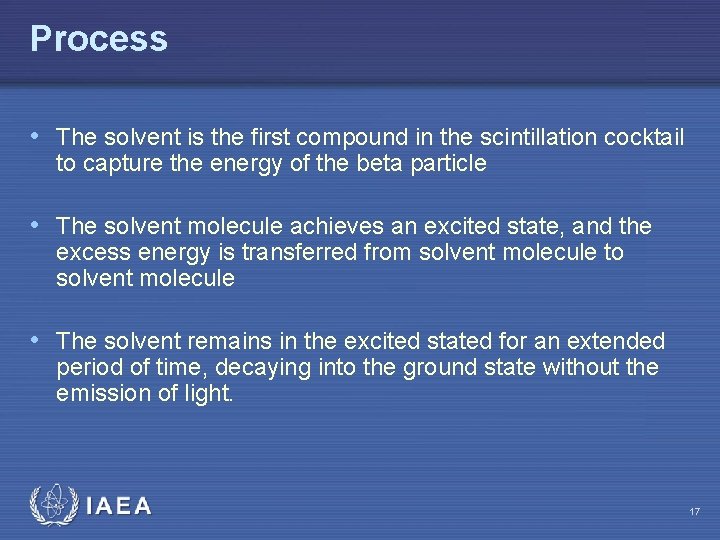
Process • The solvent is the first compound in the scintillation cocktail to capture the energy of the beta particle • The solvent molecule achieves an excited state, and the excess energy is transferred from solvent molecule to solvent molecule • The solvent remains in the excited stated for an extended period of time, decaying into the ground state without the emission of light. IAEA 17
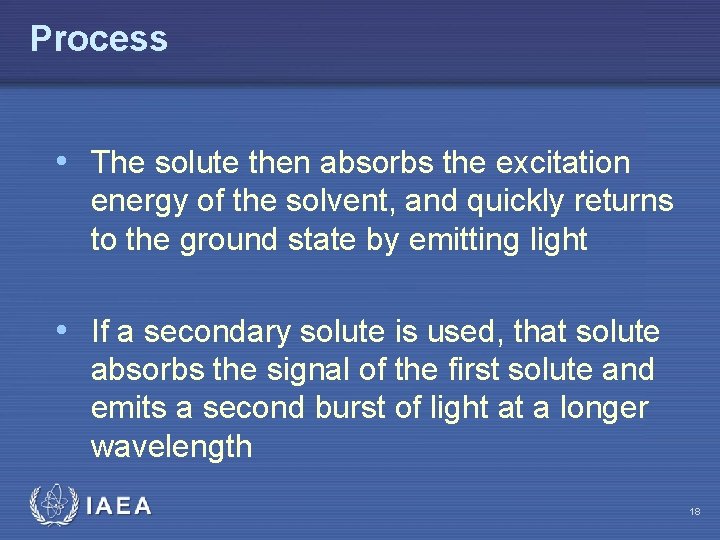
Process • The solute then absorbs the excitation energy of the solvent, and quickly returns to the ground state by emitting light • If a secondary solute is used, that solute absorbs the signal of the first solute and emits a second burst of light at a longer wavelength IAEA 18
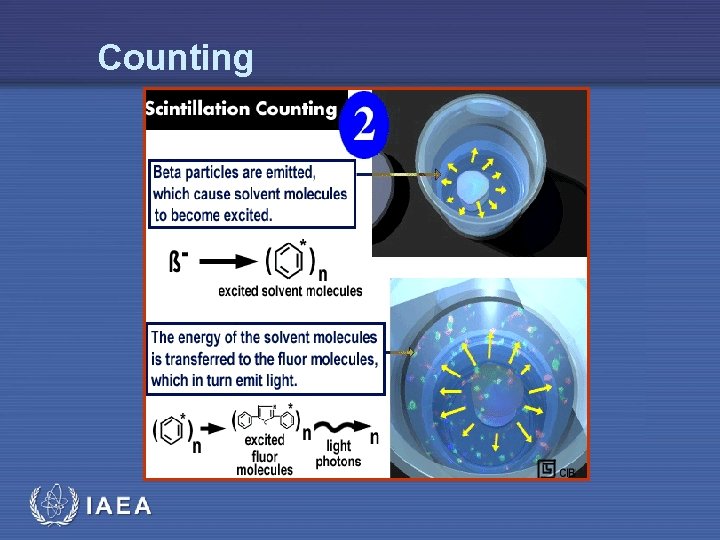
Counting IAEA
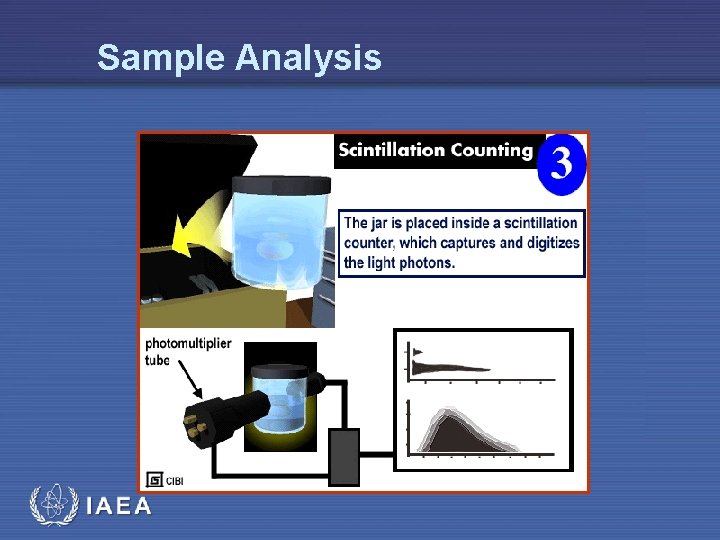
Sample Analysis IAEA
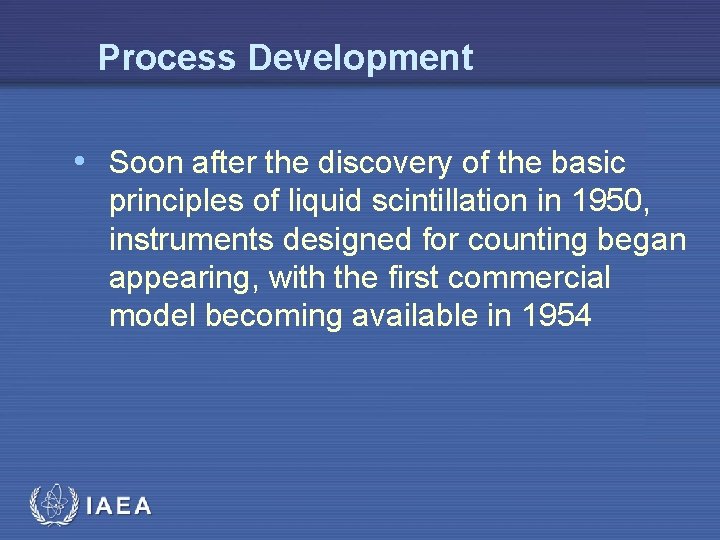
Process Development • Soon after the discovery of the basic principles of liquid scintillation in 1950, instruments designed for counting began appearing, with the first commercial model becoming available in 1954 IAEA
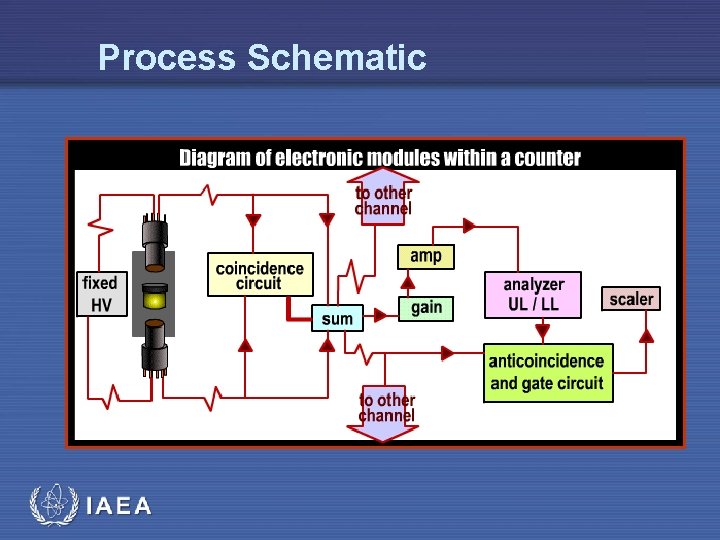
Process Schematic IAEA
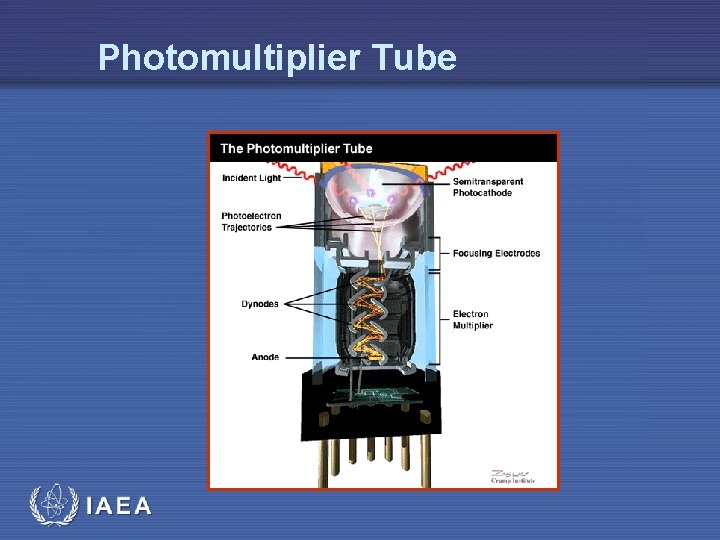
Photomultiplier Tube IAEA
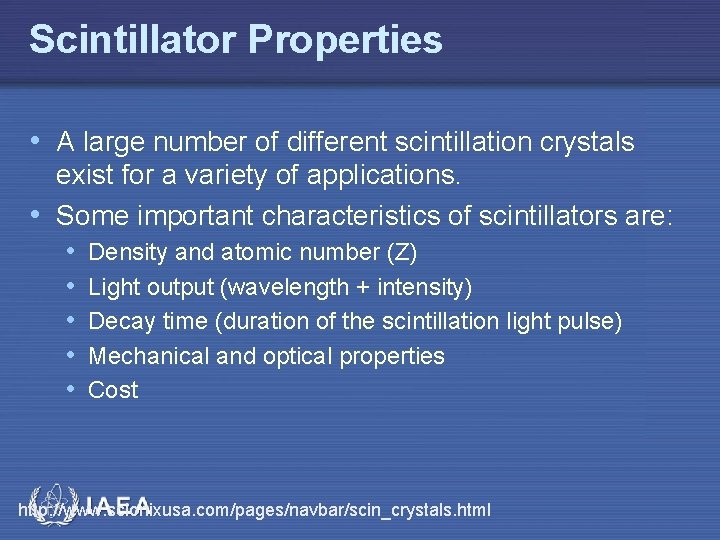
Scintillator Properties • A large number of different scintillation crystals exist for a variety of applications. • Some important characteristics of scintillators are: • Density and atomic number (Z) • Light output (wavelength + intensity) • Decay time (duration of the scintillation light pulse) • Mechanical and optical properties • Cost IAEA http: //www. scionixusa. com/pages/navbar/scin_crystals. html
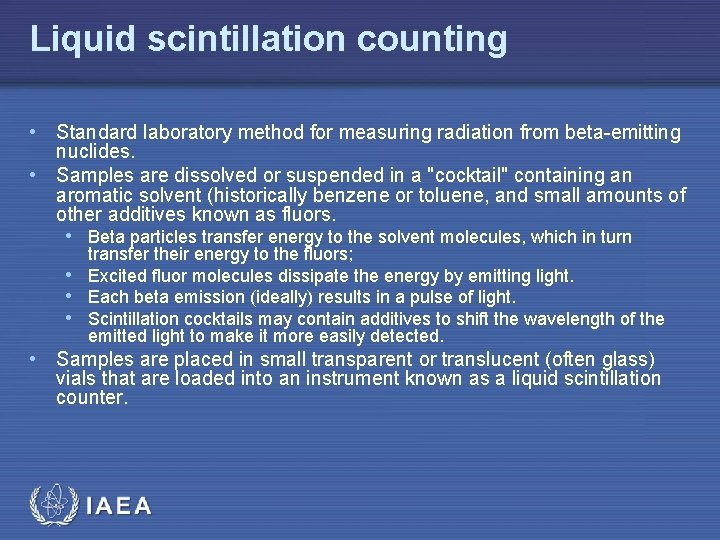
Liquid scintillation counting • Standard laboratory method for measuring radiation from beta-emitting nuclides. • Samples are dissolved or suspended in a "cocktail" containing an aromatic solvent (historically benzene or toluene, and small amounts of other additives known as fluors. • Beta particles transfer energy to the solvent molecules, which in turn transfer their energy to the fluors; • Excited fluor molecules dissipate the energy by emitting light. • Each beta emission (ideally) results in a pulse of light. • Scintillation cocktails may contain additives to shift the wavelength of the emitted light to make it more easily detected. • Samples are placed in small transparent or translucent (often glass) vials that are loaded into an instrument known as a liquid scintillation counter. IAEA
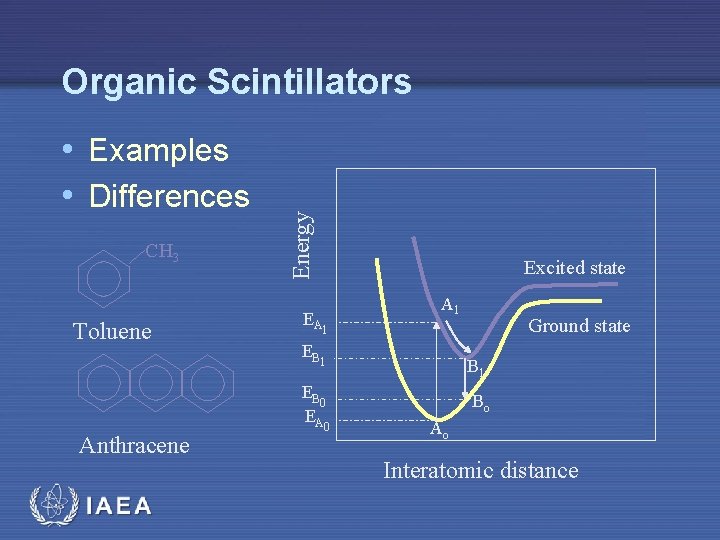
• Examples • Differences CH 3 Toluene Anthracene IAEA Energy Organic Scintillators EA 1 Excited state A 1 EB 0 EA 0 Ground state B 1 Bo Ao Interatomic distance
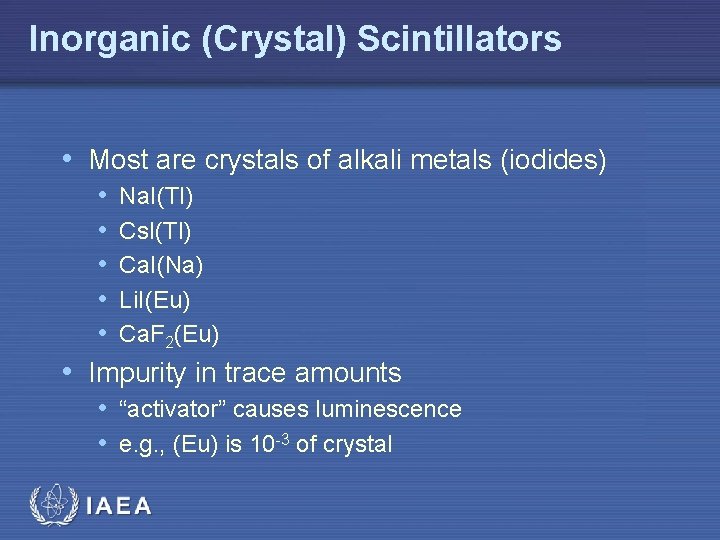
Inorganic (Crystal) Scintillators • Most are crystals of alkali metals (iodides) • Na. I(Tl) • Cs. I(Tl) • Ca. I(Na) • Li. I(Eu) • Ca. F 2(Eu) • Impurity in trace amounts • “activator” causes luminescence • e. g. , (Eu) is 10 -3 of crystal IAEA
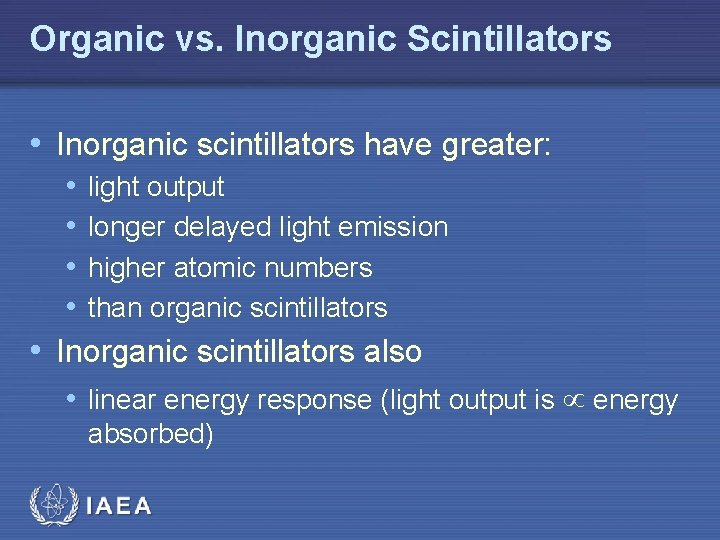
Organic vs. Inorganic Scintillators • Inorganic scintillators have greater: • light output • longer delayed light emission • higher atomic numbers • than organic scintillators • Inorganic scintillators also • linear energy response (light output is energy absorbed) IAEA
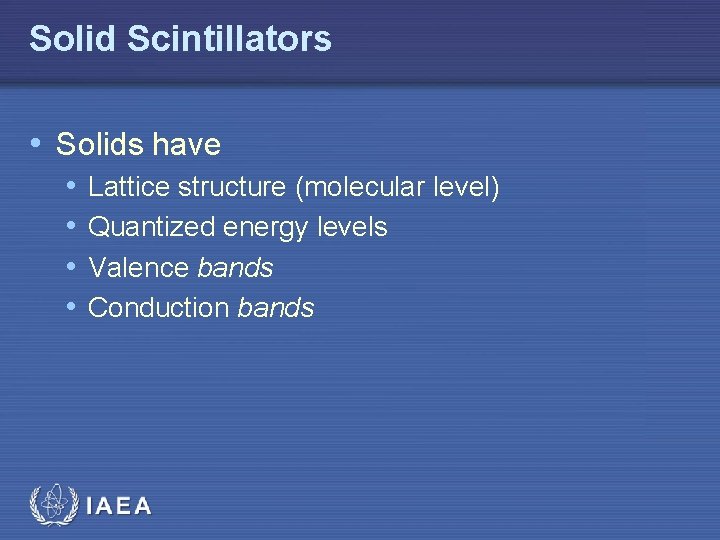
Solid Scintillators • Solids have • • Lattice structure (molecular level) Quantized energy levels Valence bands Conduction bands IAEA
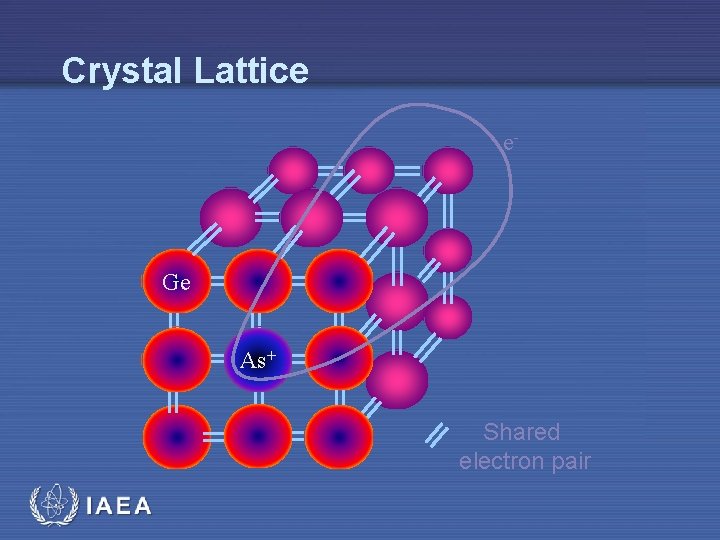
Crystal Lattice e- Ge As+ Shared electron pair IAEA
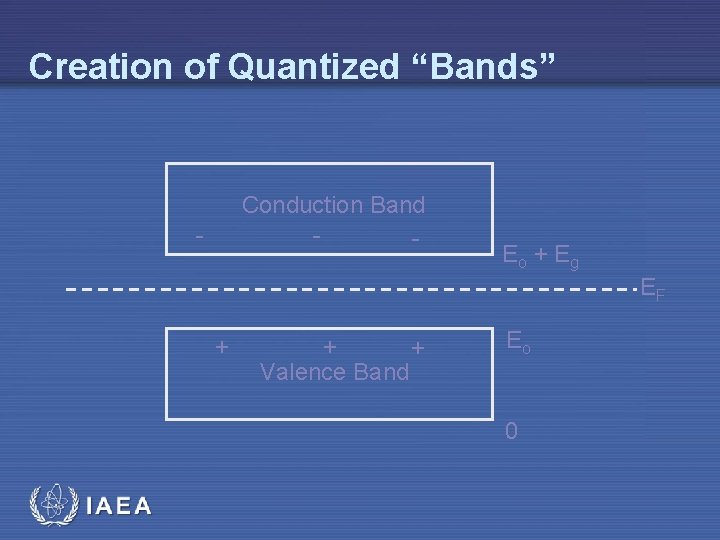
Creation of Quantized “Bands” Conduction Band - - Eo + Eg EF + + + Valence Band Eo 0 IAEA
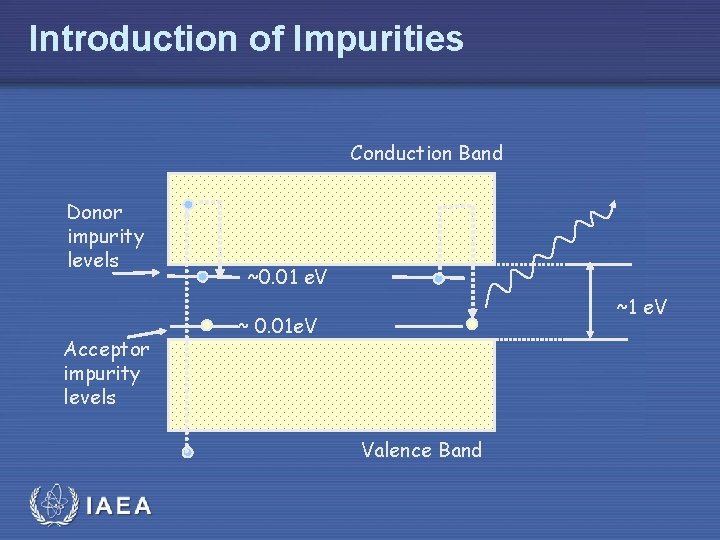
Introduction of Impurities Conduction Band Donor impurity levels Acceptor impurity levels ~0. 01 e. V ~ 0. 01 e. V Valence Band IAEA
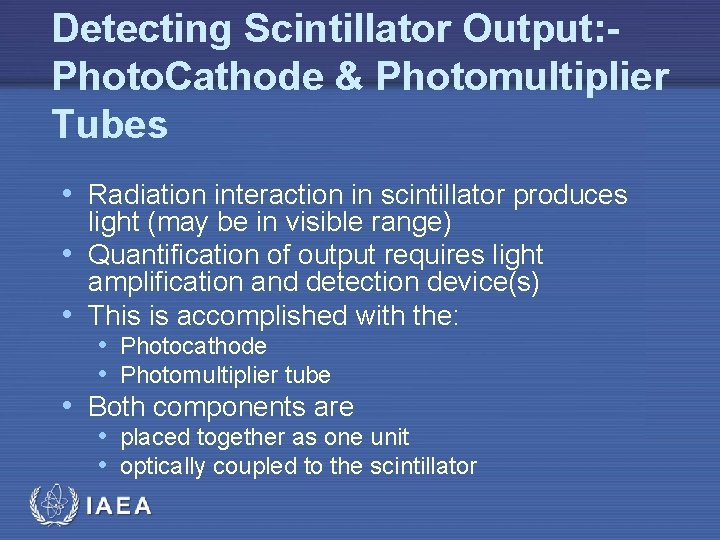
Detecting Scintillator Output: Photo. Cathode & Photomultiplier Tubes • Radiation interaction in scintillator produces light (may be in visible range) • Quantification of output requires light amplification and detection device(s) • This is accomplished with the: • Photocathode • Photomultiplier tube • Both components are • placed together as one unit • optically coupled to the scintillator IAEA
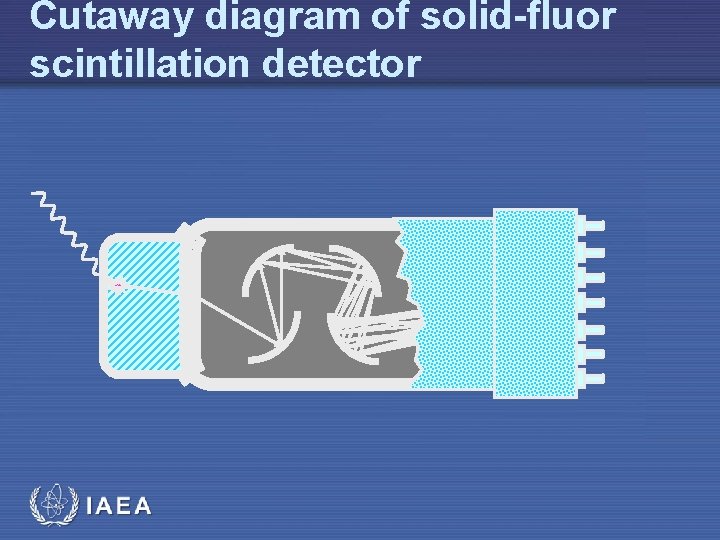
Cutaway diagram of solid-fluor scintillation detector IAEA
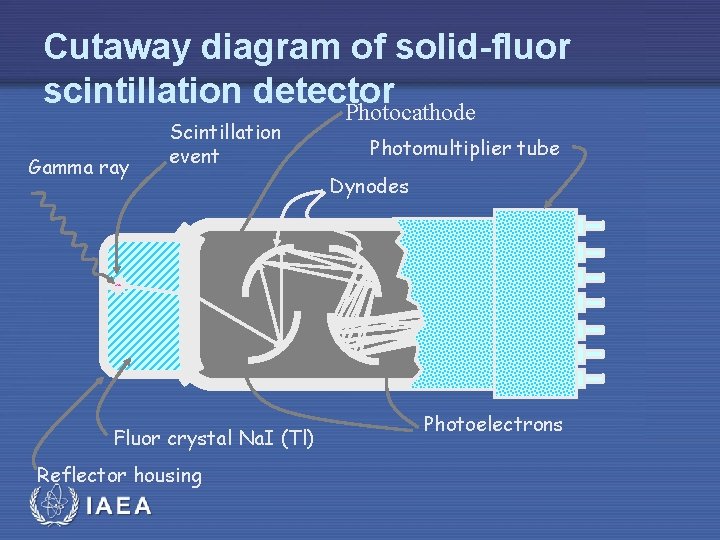
Cutaway diagram of solid-fluor scintillation detector Photocathode Gamma ray Scintillation event Fluor crystal Na. I (Tl) Reflector housing IAEA Photomultiplier tube Dynodes Photoelectrons
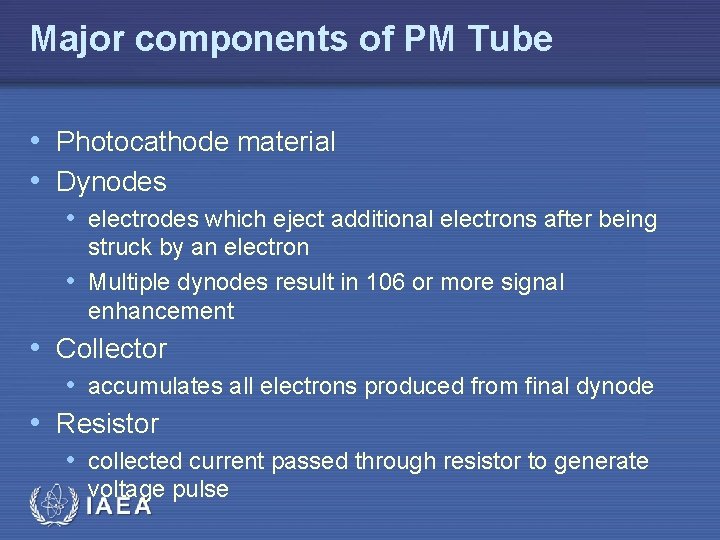
Major components of PM Tube • Photocathode material • Dynodes • electrodes which eject additional electrons after being struck by an electron • Multiple dynodes result in 106 or more signal enhancement • Collector • accumulates all electrons produced from final dynode • Resistor • collected current passed through resistor to generate voltage pulse IAEA
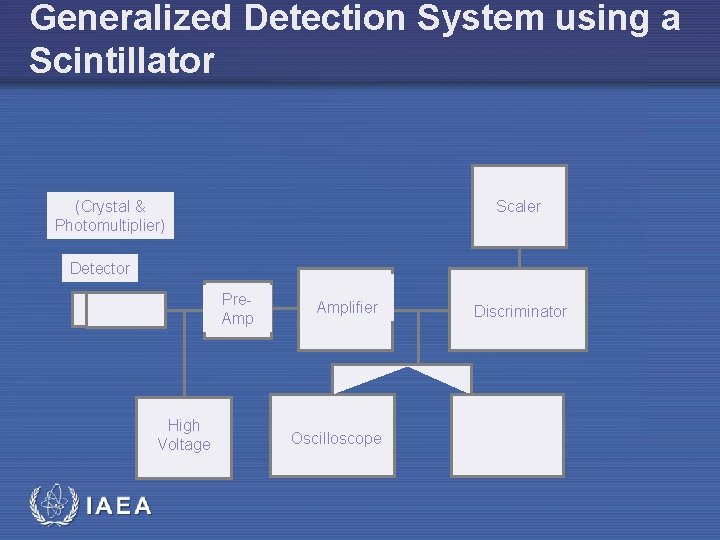
Generalized Detection System using a Scintillator (Crystal & Photomultiplier) Scaler Detector Pre. Amp High Voltage IAEA Amplifier Oscilloscope Discriminator Multi. Channel Analyzer
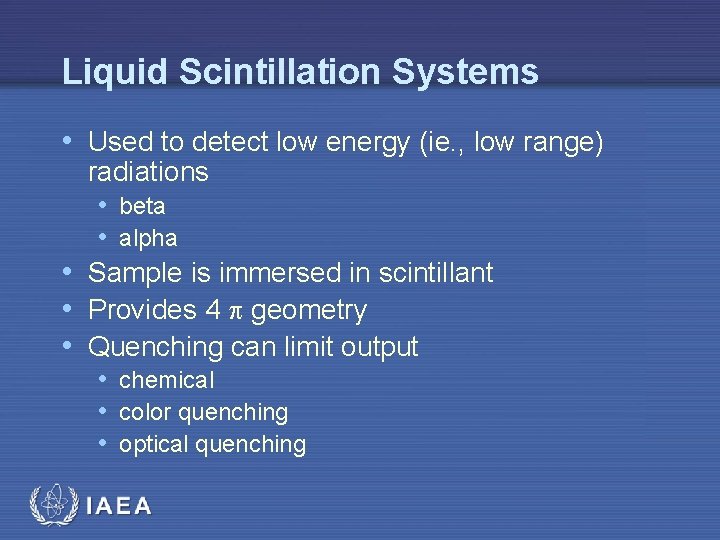
Liquid Scintillation Systems • Used to detect low energy (ie. , low range) radiations • beta • alpha • Sample is immersed in scintillant • Provides 4 geometry • Quenching can limit output • chemical • color quenching • optical quenching IAEA
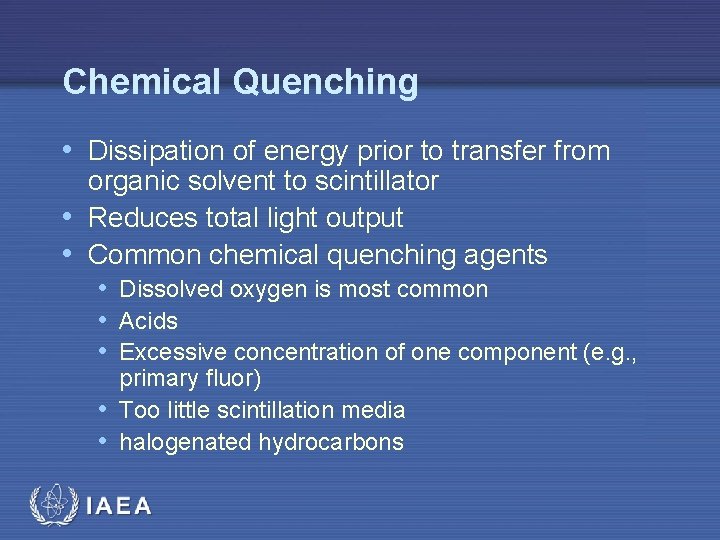
Chemical Quenching • Dissipation of energy prior to transfer from organic solvent to scintillator • Reduces total light output • Common chemical quenching agents • Dissolved oxygen is most common • Acids • Excessive concentration of one component (e. g. , primary fluor) • Too little scintillation media • halogenated hydrocarbons IAEA
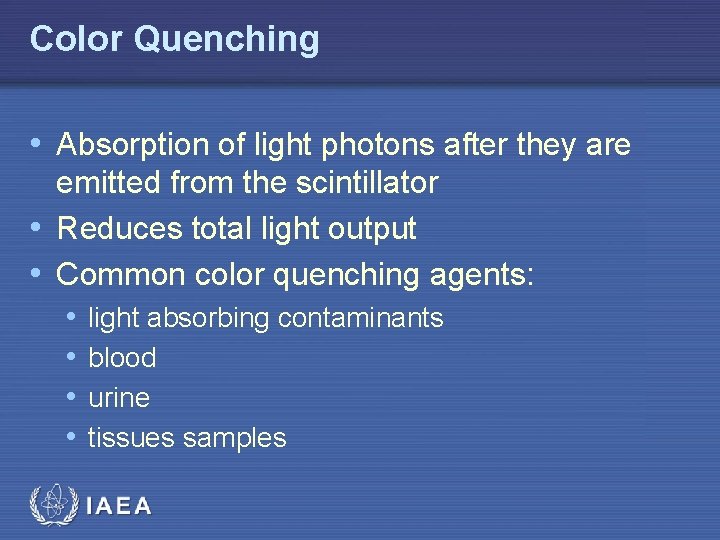
Color Quenching • Absorption of light photons after they are emitted from the scintillator • Reduces total light output • Common color quenching agents: • light absorbing contaminants • blood • urine • tissues samples IAEA
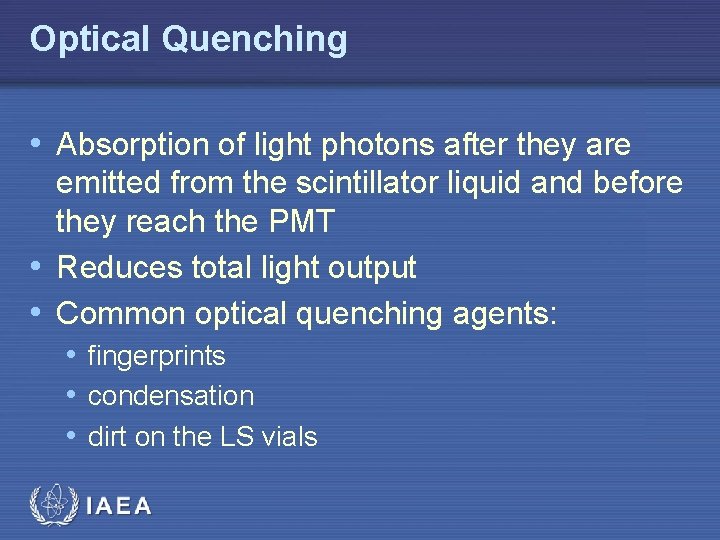
Optical Quenching • Absorption of light photons after they are emitted from the scintillator liquid and before they reach the PMT • Reduces total light output • Common optical quenching agents: • fingerprints • condensation • dirt on the LS vials IAEA
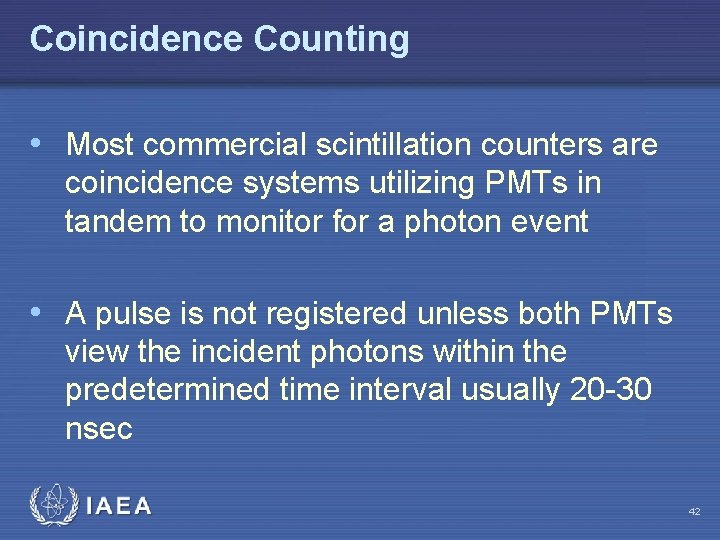
Coincidence Counting • Most commercial scintillation counters are coincidence systems utilizing PMTs in tandem to monitor for a photon event • A pulse is not registered unless both PMTs view the incident photons within the predetermined time interval usually 20 -30 nsec IAEA 42
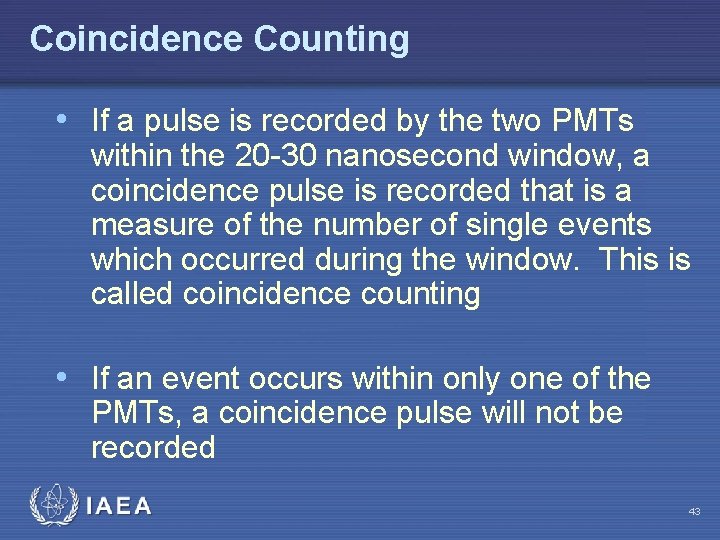
Coincidence Counting • If a pulse is recorded by the two PMTs within the 20 -30 nanosecond window, a coincidence pulse is recorded that is a measure of the number of single events which occurred during the window. This is called coincidence counting • If an event occurs within only one of the PMTs, a coincidence pulse will not be recorded IAEA 43
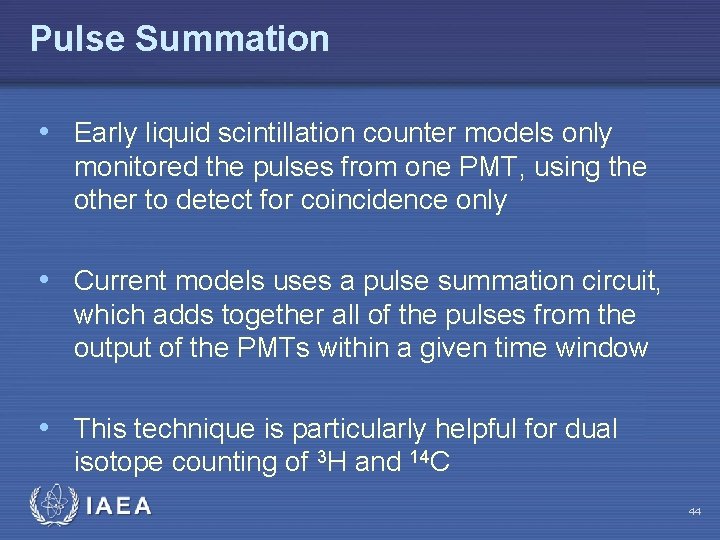
Pulse Summation • Early liquid scintillation counter models only monitored the pulses from one PMT, using the other to detect for coincidence only • Current models uses a pulse summation circuit, which adds together all of the pulses from the output of the PMTs within a given time window • This technique is particularly helpful for dual isotope counting of 3 H and 14 C IAEA 44
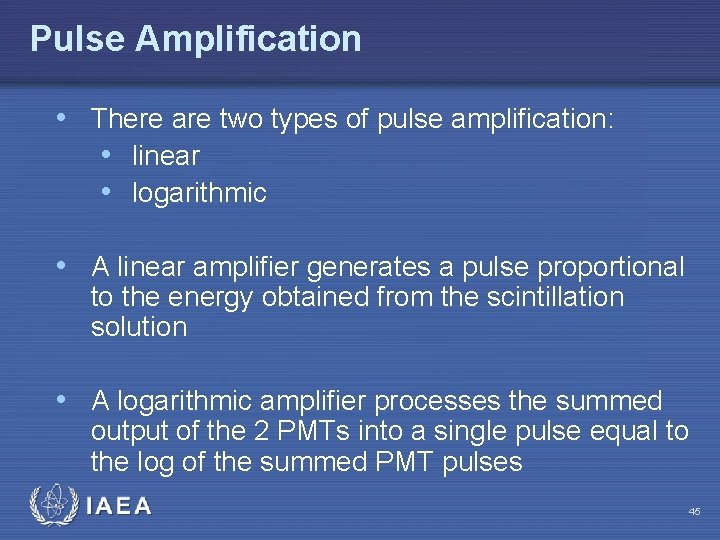
Pulse Amplification • There are two types of pulse amplification: • linear • logarithmic • A linear amplifier generates a pulse proportional to the energy obtained from the scintillation solution • A logarithmic amplifier processes the summed output of the 2 PMTs into a single pulse equal to the log of the summed PMT pulses IAEA 45
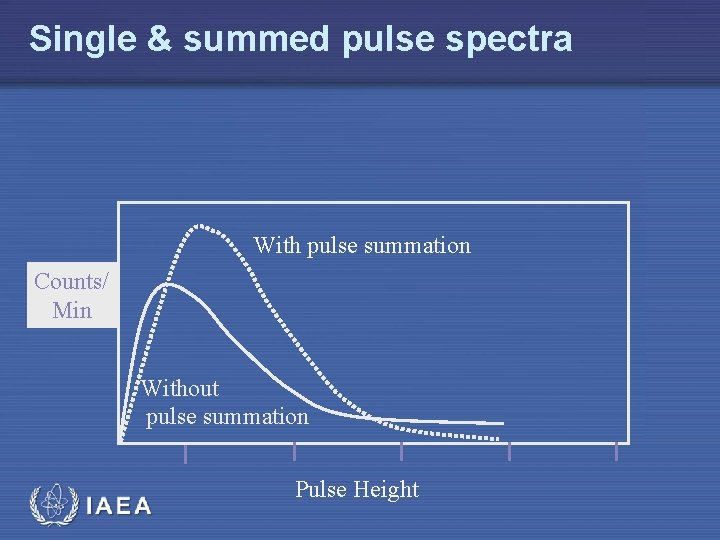
Single & summed pulse spectra With pulse summation Counts/ Min Without pulse summation IAEA Pulse Height
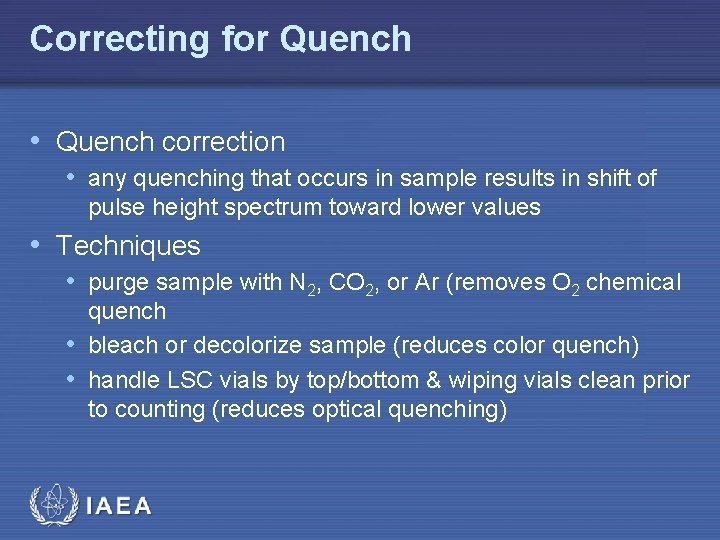
Correcting for Quench • Quench correction • any quenching that occurs in sample results in shift of pulse height spectrum toward lower values • Techniques • purge sample with N 2, CO 2, or Ar (removes O 2 chemical quench • bleach or decolorize sample (reduces color quench) • handle LSC vials by top/bottom & wiping vials clean prior to counting (reduces optical quenching) IAEA
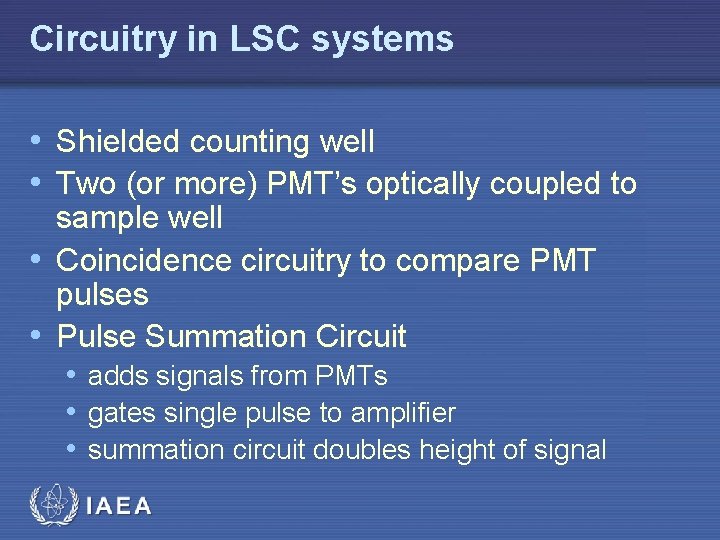
Circuitry in LSC systems • Shielded counting well • Two (or more) PMT’s optically coupled to sample well • Coincidence circuitry to compare PMT pulses • Pulse Summation Circuit • adds signals from PMTs • gates single pulse to amplifier • summation circuit doubles height of signal IAEA
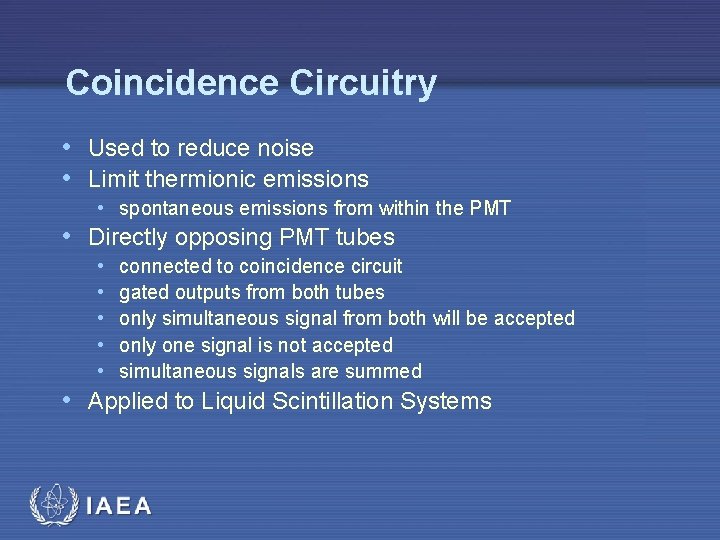
Coincidence Circuitry • Used to reduce noise • Limit thermionic emissions • spontaneous emissions from within the PMT • Directly opposing PMT tubes • • • connected to coincidence circuit gated outputs from both tubes only simultaneous signal from both will be accepted only one signal is not accepted simultaneous signals are summed • Applied to Liquid Scintillation Systems IAEA
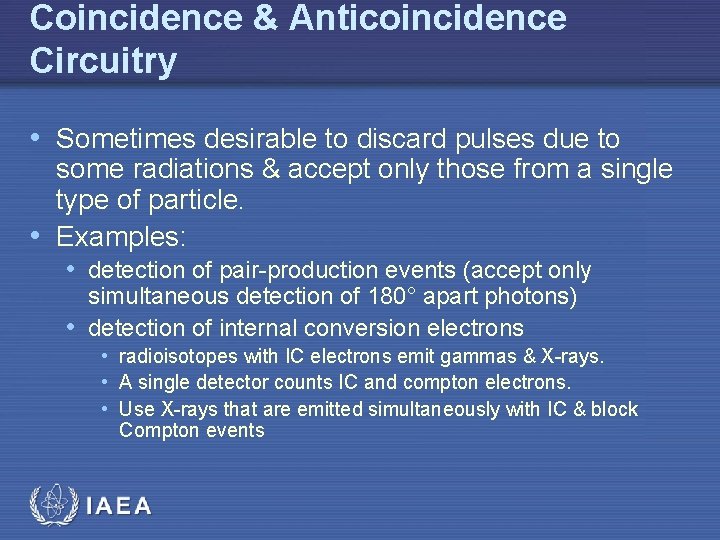
Coincidence & Anticoincidence Circuitry • Sometimes desirable to discard pulses due to some radiations & accept only those from a single type of particle. • Examples: • detection of pair-production events (accept only simultaneous detection of 180° apart photons) • detection of internal conversion electrons • radioisotopes with IC electrons emit gammas & X-rays. • A single detector counts IC and compton electrons. • Use X-rays that are emitted simultaneously with IC & block Compton events IAEA
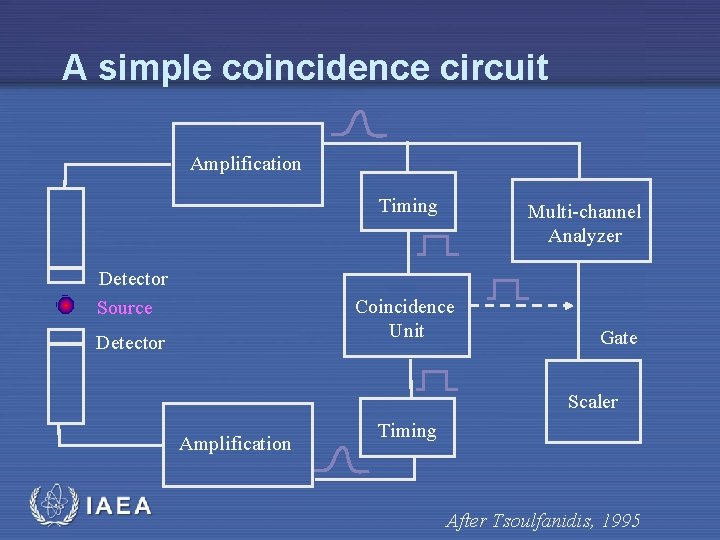
A simple coincidence circuit Amplification Timing Detector Source Multi-channel Analyzer Coincidence Unit Detector Gate Scaler Amplification IAEA Timing After Tsoulfanidis, 1995
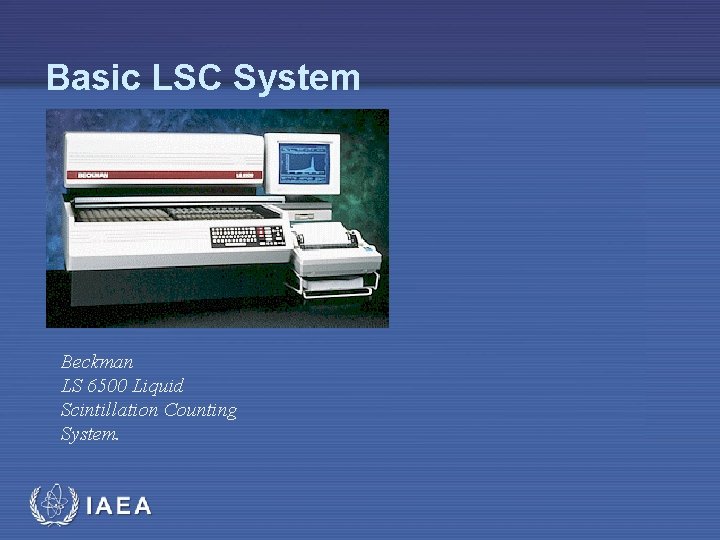
Basic LSC System Beckman LS 6500 Liquid Scintillation Counting System. IAEA
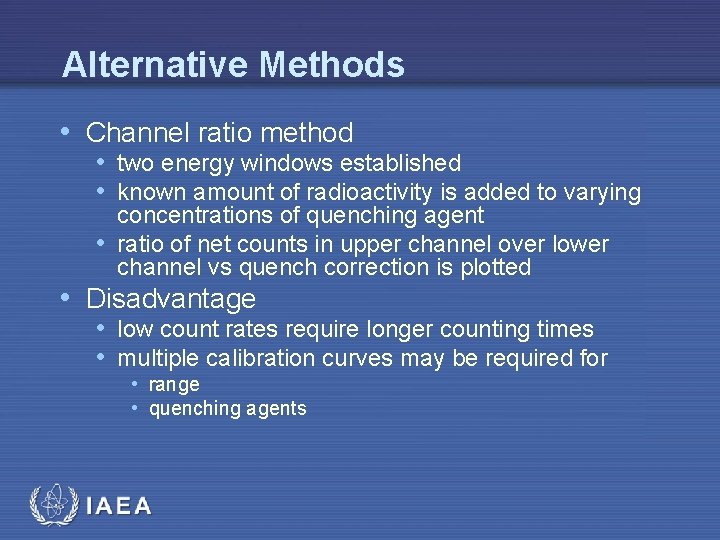
Alternative Methods • Channel ratio method • two energy windows established • known amount of radioactivity is added to varying concentrations of quenching agent • ratio of net counts in upper channel over lower channel vs quench correction is plotted • Disadvantage • low count rates require longer counting times • multiple calibration curves may be required for • range • quenching agents IAEA
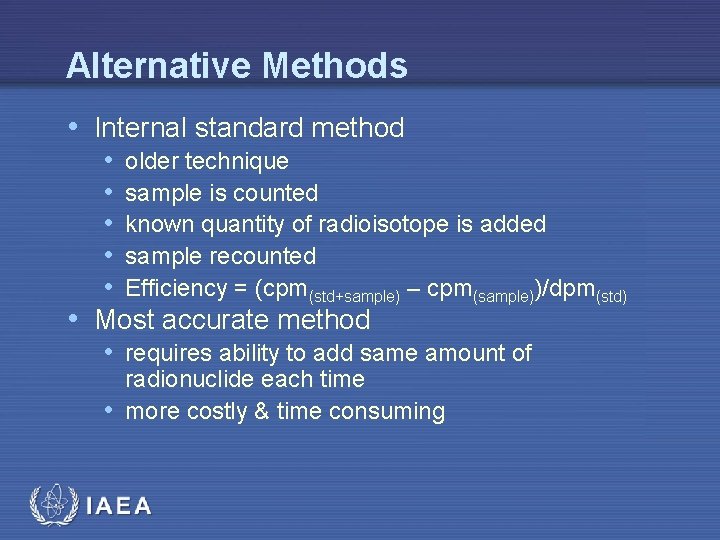
Alternative Methods • Internal standard method • older technique • sample is counted • known quantity of radioisotope is added • sample recounted • Efficiency = (cpm(std+sample) – cpm(sample))/dpm(std) • Most accurate method • requires ability to add same amount of radionuclide each time • more costly & time consuming IAEA
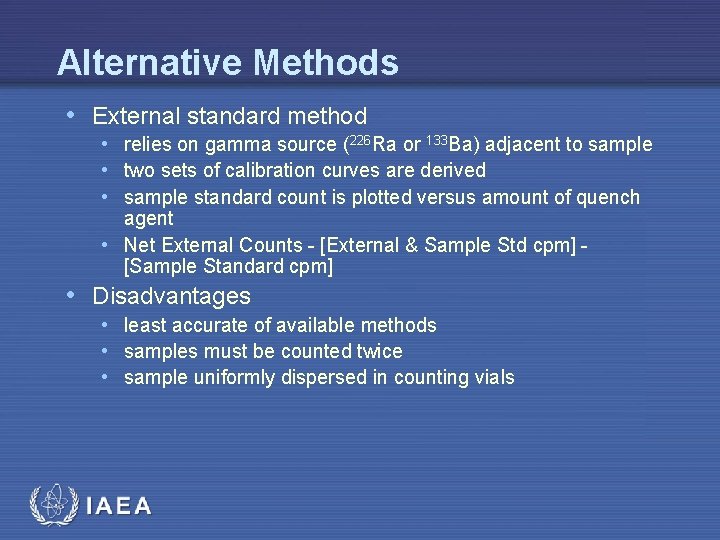
Alternative Methods • External standard method • relies on gamma source (226 Ra or 133 Ba) adjacent to sample • two sets of calibration curves are derived • sample standard count is plotted versus amount of quench agent • Net External Counts - [External & Sample Std cpm] - [Sample Standard cpm] • Disadvantages • least accurate of available methods • samples must be counted twice • sample uniformly dispersed in counting vials IAEA
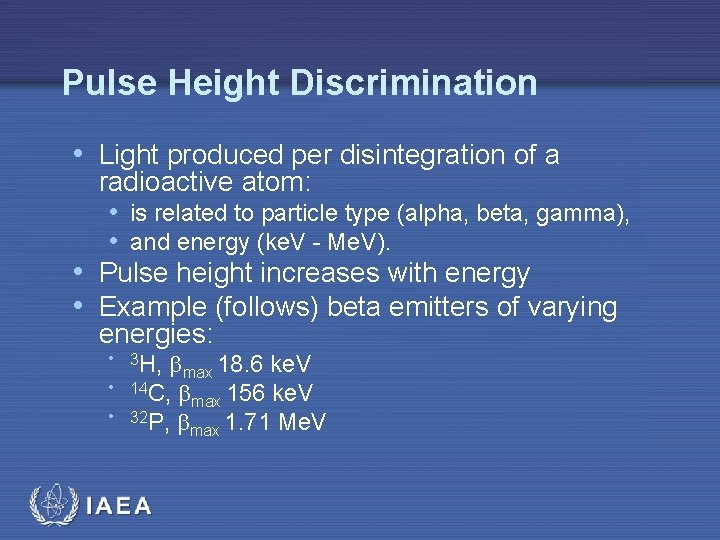
Pulse Height Discrimination • Light produced per disintegration of a radioactive atom: • is related to particle type (alpha, beta, gamma), • and energy (ke. V - Me. V). • Pulse height increases with energy • Example (follows) beta emitters of varying energies: • 3 H, max 18. 6 ke. V • 14 C, • 32 P, IAEA max 156 ke. V max 1. 71 Me. V
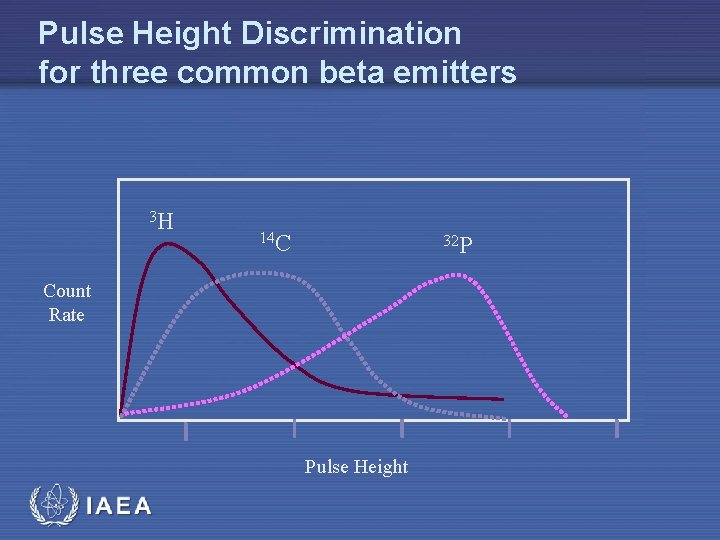
Pulse Height Discrimination for three common beta emitters 3 H 14 C 32 P Count Rate Pulse Height IAEA
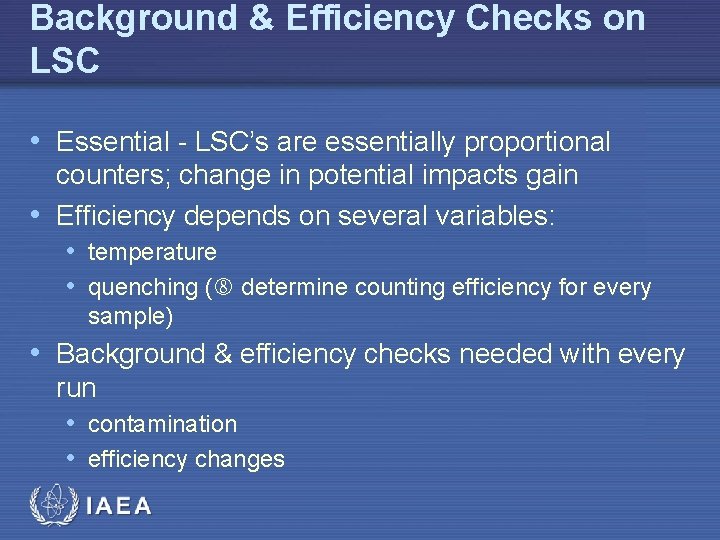
Background & Efficiency Checks on LSC • Essential - LSC’s are essentially proportional counters; change in potential impacts gain • Efficiency depends on several variables: • temperature • quenching ( determine counting efficiency for every sample) • Background & efficiency checks needed with every run • contamination • efficiency changes IAEA
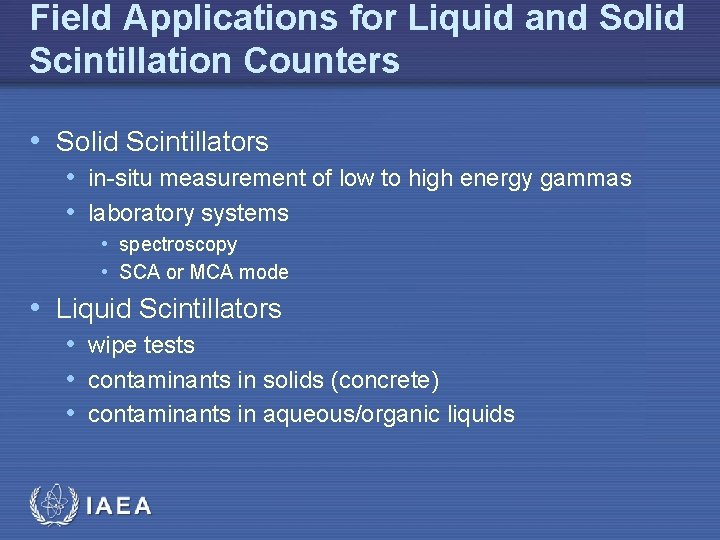
Field Applications for Liquid and Solid Scintillation Counters • Solid Scintillators • in-situ measurement of low to high energy gammas • laboratory systems • spectroscopy • SCA or MCA mode • Liquid Scintillators • wipe tests • contaminants in solids (concrete) • contaminants in aqueous/organic liquids IAEA
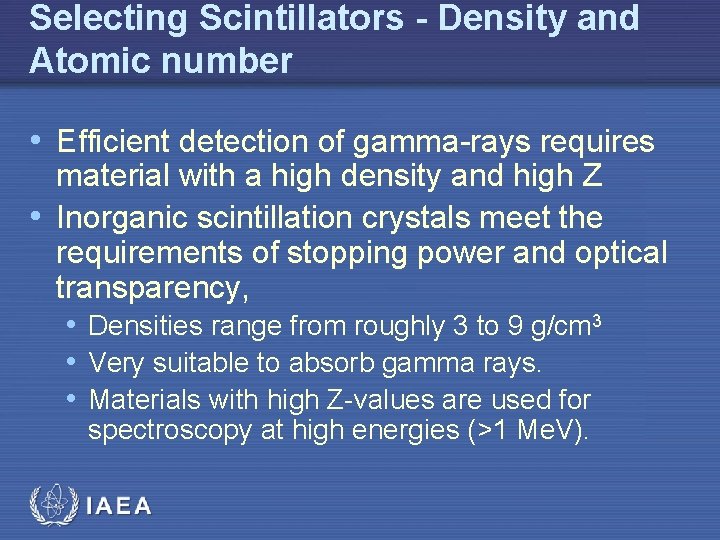
Selecting Scintillators - Density and Atomic number • Efficient detection of gamma-rays requires material with a high density and high Z • Inorganic scintillation crystals meet the requirements of stopping power and optical transparency, • Densities range from roughly 3 to 9 g/cm 3 • Very suitable to absorb gamma rays. • Materials with high Z-values are used for spectroscopy at high energies (>1 Me. V). IAEA
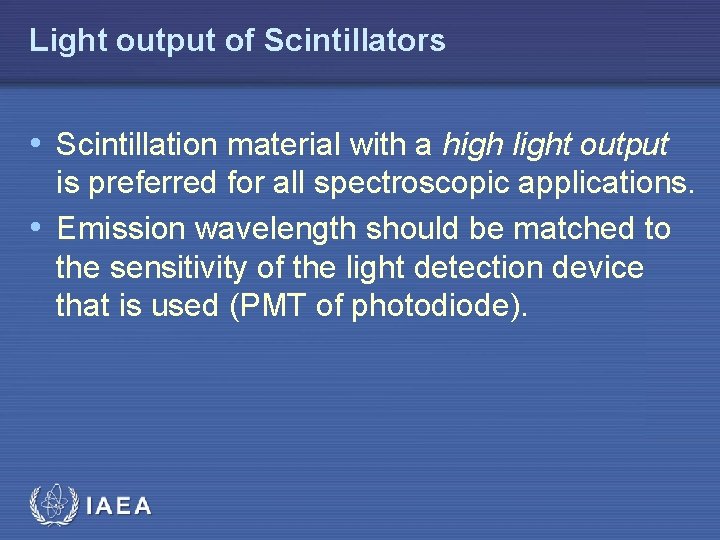
Light output of Scintillators • Scintillation material with a high light output is preferred for all spectroscopic applications. • Emission wavelength should be matched to the sensitivity of the light detection device that is used (PMT of photodiode). IAEA
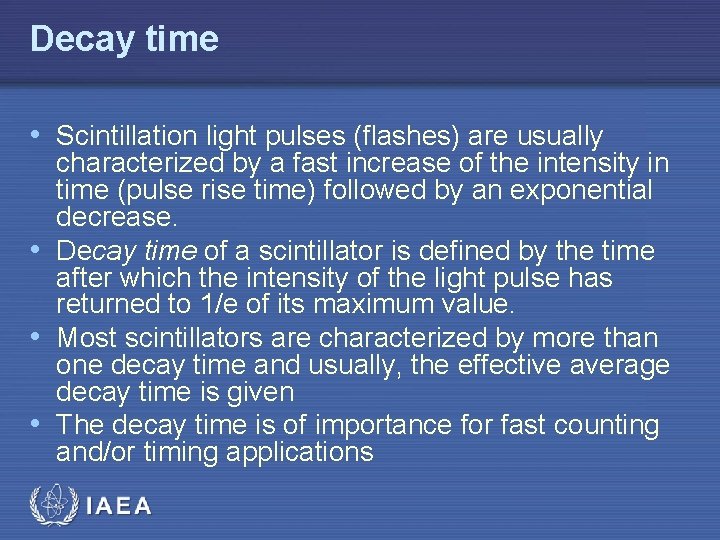
Decay time • Scintillation light pulses (flashes) are usually characterized by a fast increase of the intensity in time (pulse rise time) followed by an exponential decrease. • Decay time of a scintillator is defined by the time after which the intensity of the light pulse has returned to 1/e of its maximum value. • Most scintillators are characterized by more than one decay time and usually, the effective average decay time is given • The decay time is of importance for fast counting and/or timing applications IAEA
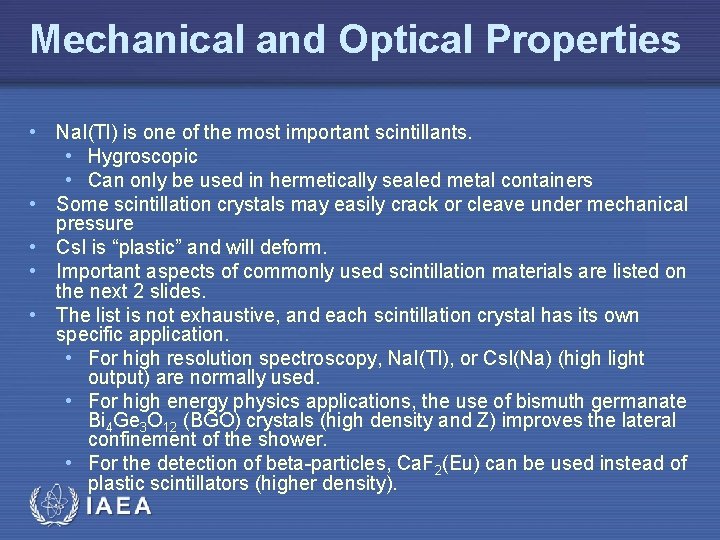
Mechanical and Optical Properties • Na. I(Tl) is one of the most important scintillants. • Hygroscopic • Can only be used in hermetically sealed metal containers • Some scintillation crystals may easily crack or cleave under mechanical pressure • Cs. I is “plastic” and will deform. • Important aspects of commonly used scintillation materials are listed on the next 2 slides. • The list is not exhaustive, and each scintillation crystal has its own specific application. • For high resolution spectroscopy, Na. I(Tl), or Cs. I(Na) (high light output) are normally used. • For high energy physics applications, the use of bismuth germanate Bi 4 Ge 3 O 12 (BGO) crystals (high density and Z) improves the lateral confinement of the shower. • For the detection of beta-particles, Ca. F 2(Eu) can be used instead of plastic scintillators (higher density). IAEA
![Commonly Used Scintillators Density gcm 3 Emission Max nm Decay Constant 1 Refractive Index Commonly Used Scintillators Density [g/cm 3] Emission Max [nm] Decay Constant (1) Refractive Index](https://slidetodoc.com/presentation_image/7e3d3ae3479150717d2fd9fb070c1b0f/image-64.jpg)
Commonly Used Scintillators Density [g/cm 3] Emission Max [nm] Decay Constant (1) Refractive Index (2) Conversion Efficiency (3) Hygroscopic Na. I(Tl) 3. 67 415 0. 23 ms 1. 85 100 yes Cs. I(Tl) 4. 51 550 0. 6/3. 4 ms 1. 79 45 no Cs. I(Na) 4. 51 420 0. 63 ms 1. 84 85 slightly Cs. I undoped 4. 51 315 16 ns 1. 95 4 - 6 no Ca. F 2 (Eu) 3. 18 435 0. 84 ms 1. 47 50 no 6 Li. I (Eu) 4. 08 470 1. 4 ms 1. 96 35 yes 2. 6 390 - 430 60 ns 1. 56 4 - 6 no 4. 64 390 3 - 5 ns 1. 48 5 - 7 yes Material 6 Li - glass Cs. F IAEA (1) Effective average decay time For g-rays. (2) At the wavelength of the emission maximum. (3) Relative scintillation signal at room temperature for g-rays when coupled to a photomultiplier tube with a Bi-Alkalai photocathode.
![Commonly Used Scintillators Material Density gcm 3 Emission Maximum nm Decay Constant 1 Refractive Commonly Used Scintillators Material Density [g/cm 3] Emission Maximum [nm] Decay Constant (1) Refractive](https://slidetodoc.com/presentation_image/7e3d3ae3479150717d2fd9fb070c1b0f/image-65.jpg)
Commonly Used Scintillators Material Density [g/cm 3] Emission Maximum [nm] Decay Constant (1) Refractive Index (2) Conversion Efficiency (3) Hygros copic Ba. F 2 4. 88 315 220 0. 63 ms 0. 8 ns 1. 50 1. 54 16 5 no YAP (Ce) 5. 55 350 27 ns 1. 94 35 - 40 no GSO (Ce) 6. 71 440 30 - 60 ns 1. 85 20 - 25 no BGO 7. 13 480 0. 3 ms 2. 15 15 - 20 no Cd. WO 4 7. 90 470 / 540 20 / 5 ms 2. 3 25 - 30 no Plastics 1. 03 375 - 600 1 - 3 ms 1. 58 25 - 30 no (1) Effective agerage decay time For g-rays. (2) At the wavelength of the emission maximum. (3) Relative scintillation signal at room temperature for g-rays when coupled to a photomultiplier tube with a Bi-Alkalai photocathode. IAEA
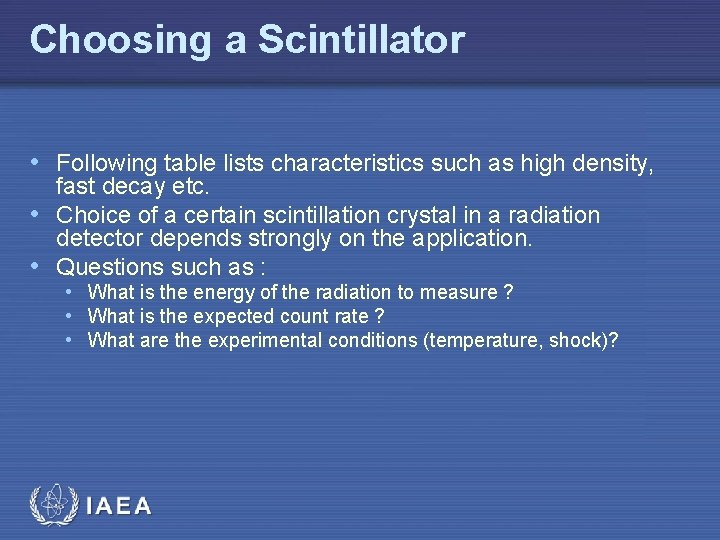
Choosing a Scintillator • Following table lists characteristics such as high density, fast decay etc. • Choice of a certain scintillation crystal in a radiation detector depends strongly on the application. • Questions such as : • What is the energy of the radiation to measure ? • What is the expected count rate ? • What are the experimental conditions (temperature, shock)? IAEA
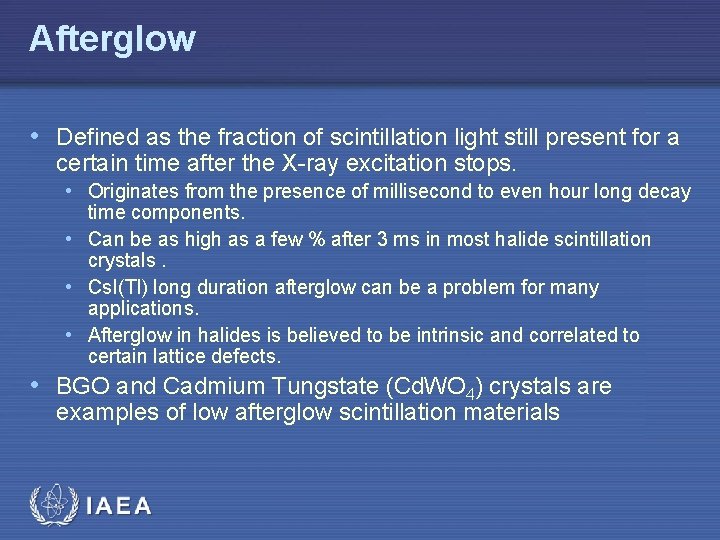
Afterglow • Defined as the fraction of scintillation light still present for a certain time after the X-ray excitation stops. • Originates from the presence of millisecond to even hour long decay time components. • Can be as high as a few % after 3 ms in most halide scintillation crystals. • Cs. I(Tl) long duration afterglow can be a problem for many applications. • Afterglow in halides is believed to be intrinsic and correlated to certain lattice defects. • BGO and Cadmium Tungstate (Cd. WO 4) crystals are examples of low afterglow scintillation materials IAEA
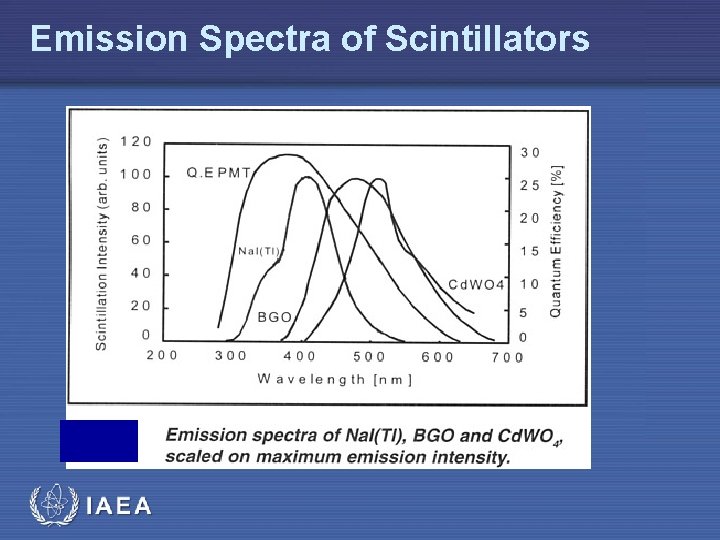
Emission Spectra of Scintillators IAEA
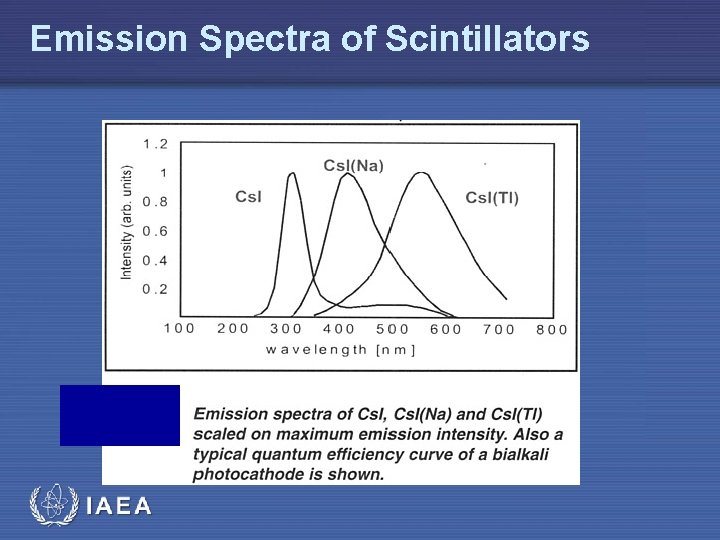
Emission Spectra of Scintillators IAEA
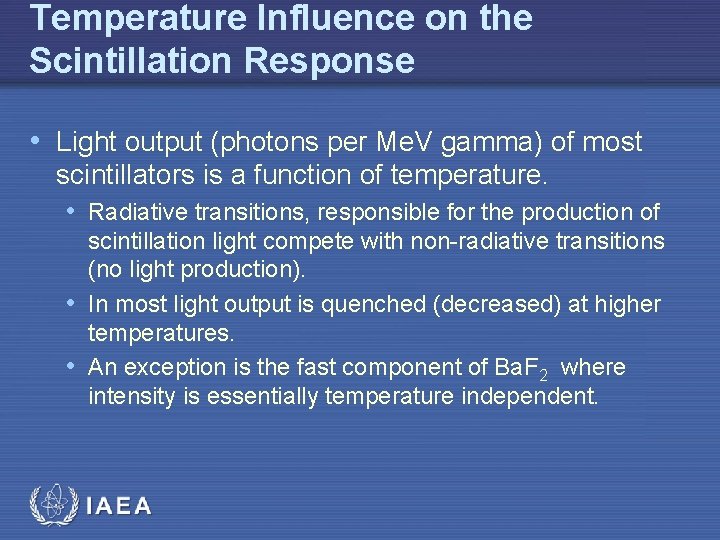
Temperature Influence on the Scintillation Response • Light output (photons per Me. V gamma) of most scintillators is a function of temperature. • Radiative transitions, responsible for the production of scintillation light compete with non-radiative transitions (no light production). • In most light output is quenched (decreased) at higher temperatures. • An exception is the fast component of Ba. F 2 where intensity is essentially temperature independent. IAEA
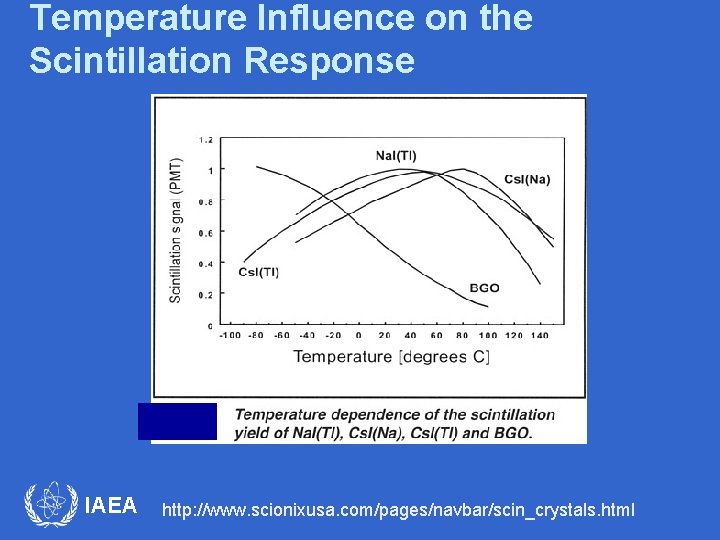
Temperature Influence on the Scintillation Response IAEA http: //www. scionixusa. com/pages/navbar/scin_crystals. html
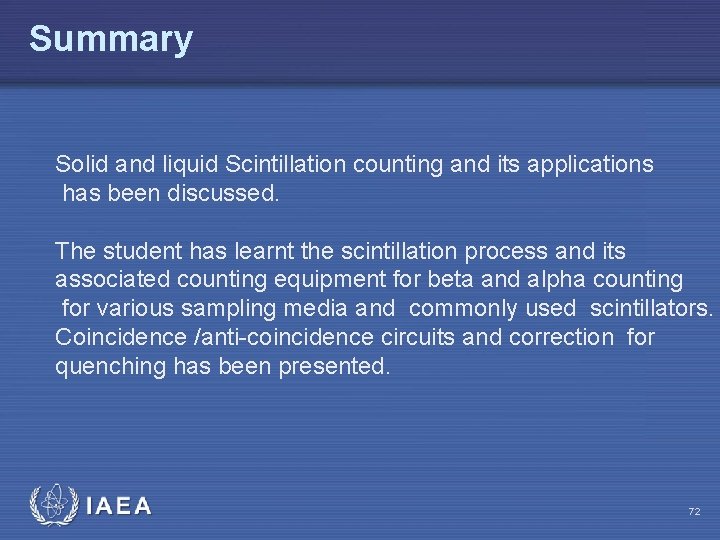
Summary Solid and liquid Scintillation counting and its applications has been discussed. The student has learnt the scintillation process and its associated counting equipment for beta and alpha counting for various sampling media and commonly used scintillators. Coincidence /anti-coincidence circuits and correction for quenching has been presented. IAEA 72
Radiation protection officer qualifications
Barium sulphate board for radiation protection
Cardinal principles of radiation protection
National radiation protection authority namibia
Iaea ssr-5
Saris iaea
Iaea livechart
Intouch plus iaea
Iaea gsr part 4
Rtc protection film
Nuclear wastes
Iaea
Stefano monti iaea
Iaea
Iaea
Iaea
Iaea
Iaea
Gsr part 7
Pcmf
Film badge
Iaea
Gsr part 2
For educational purposes only
Course number and title
Post merger integration course
One brick t junction in english bond
Chaine parallèle muscle
Ogaps
Nature meaning and scope of psychology
Conduction or convection
Greenhouse effect long and shortwave radiation
Solid and liquid venn diagram
For parents and friends radiation threat
Ionizing radiation examples
What is heat transfer conduction convection and radiation
Examples of radiation
Radiation integrals and auxiliary potential functions
Cyclotron and synchrotron radiation
Venn diagram of conduction, convection and radiation
Whats a convection current
Radiation travels in straight line. (true/false)
Radiation examples
Convection conduction radiation
Convection examples
Radiation and dna
How are conduction convection and radiation alike
How
Method of heat transfer
Conduction examples
Zeta phi beta graduate chapter intake process
Gss fast stream
Graduate success attributes
Joseph wibowo hadipoespito
Graduate success attributes utm
Lsu thesis and dissertation
Graduate college jamshedpur
Aldirecruitment.co.uk
15-780 graduate artificial intelligence
School of graduate studies upm
Pfizer uk graduate scheme
Navy voled
15-780 graduate artificial intelligence
Undergraduate graduate postgraduate
Hamline university graduate programs
Graduate school korea university
Georgia requirements for high school graduation
Usf admission portal
Bis graduate program
Graduate institute of electronics engineering
Nau off campus housing
Nauget
Uws graduate attributes