Researches and applied measurements on nuclear physics and
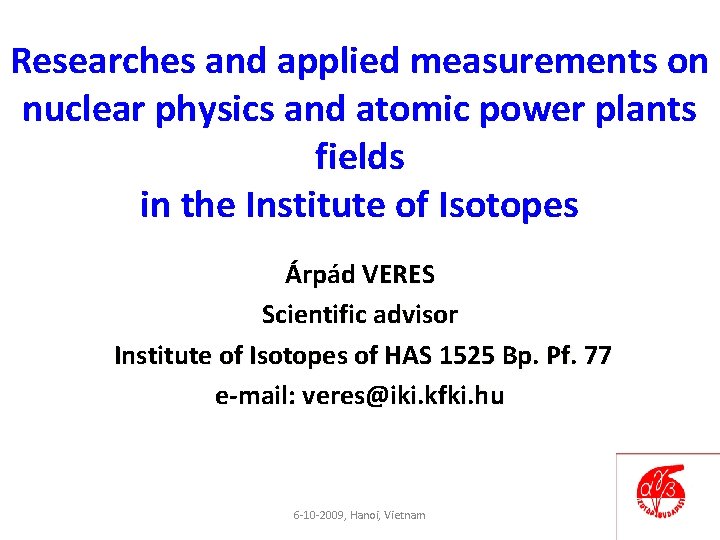
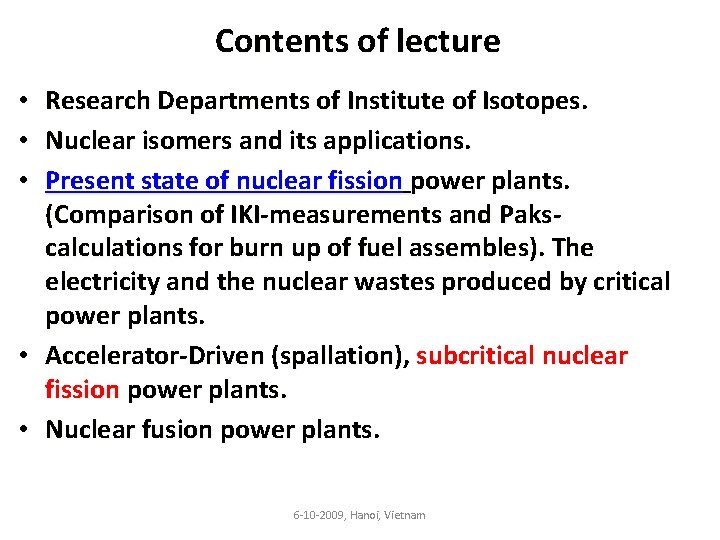
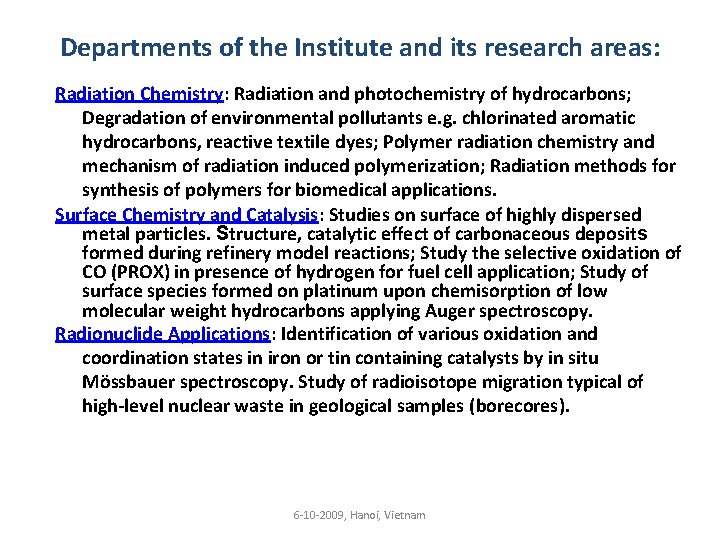
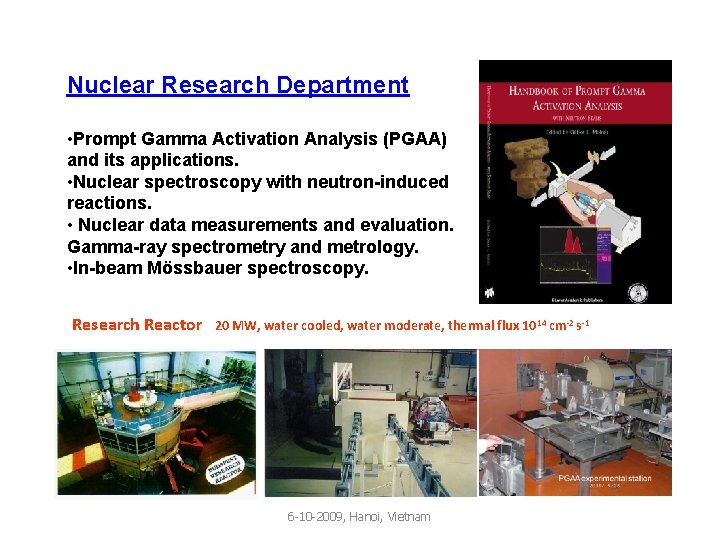
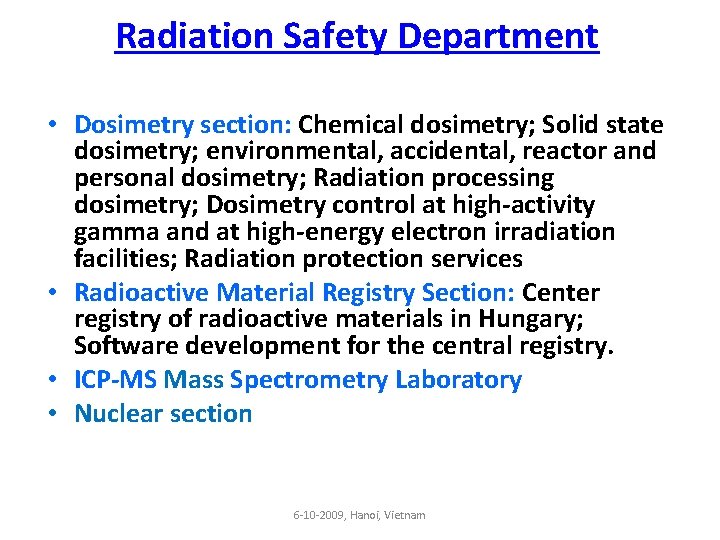
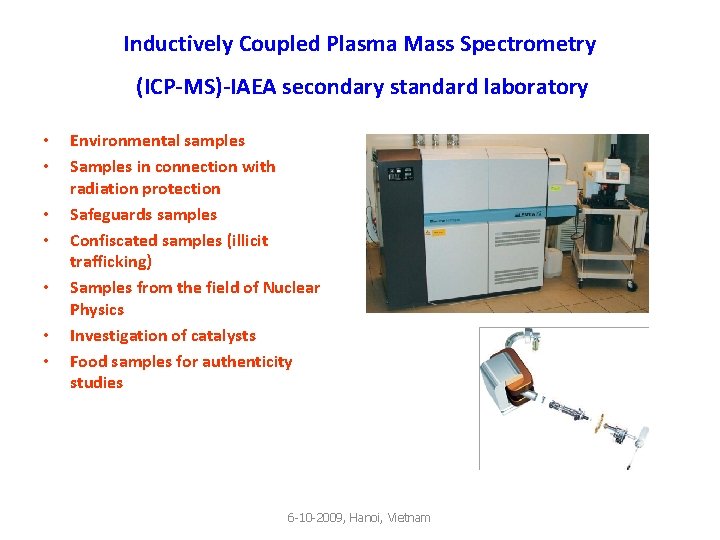
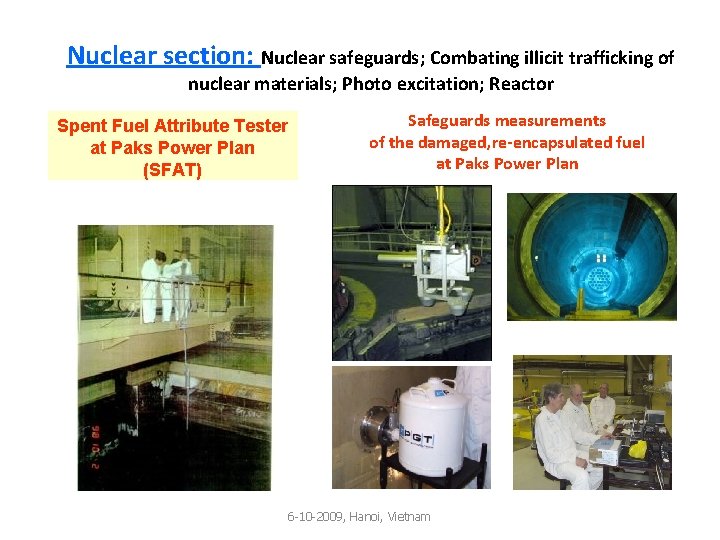
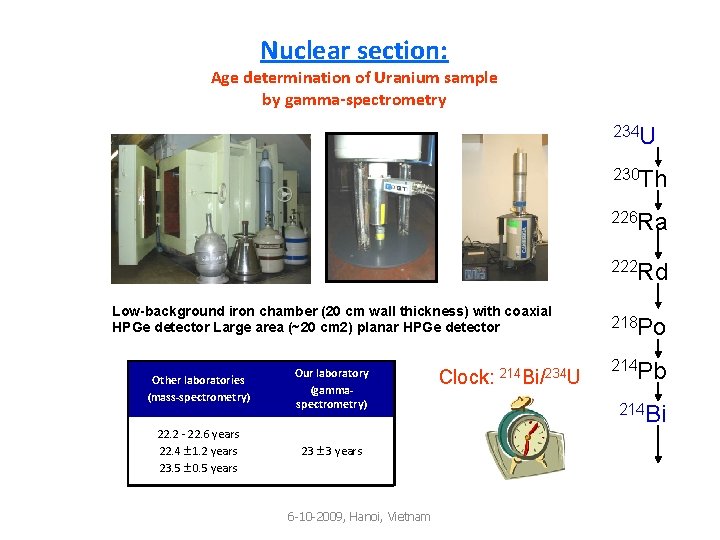
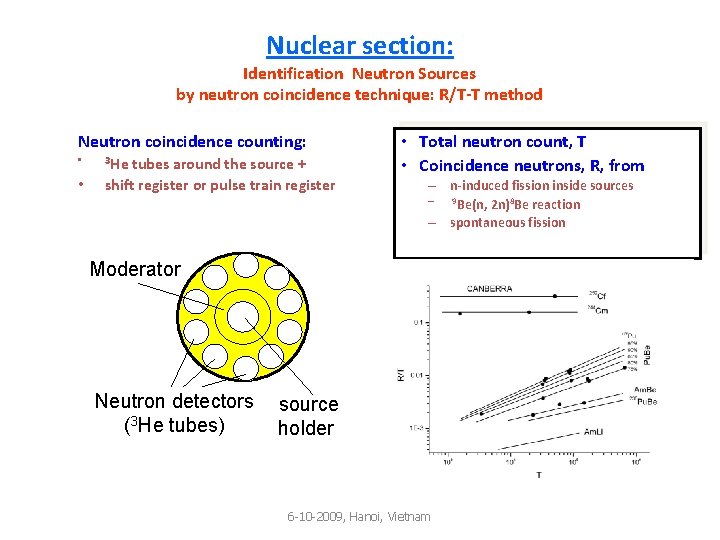
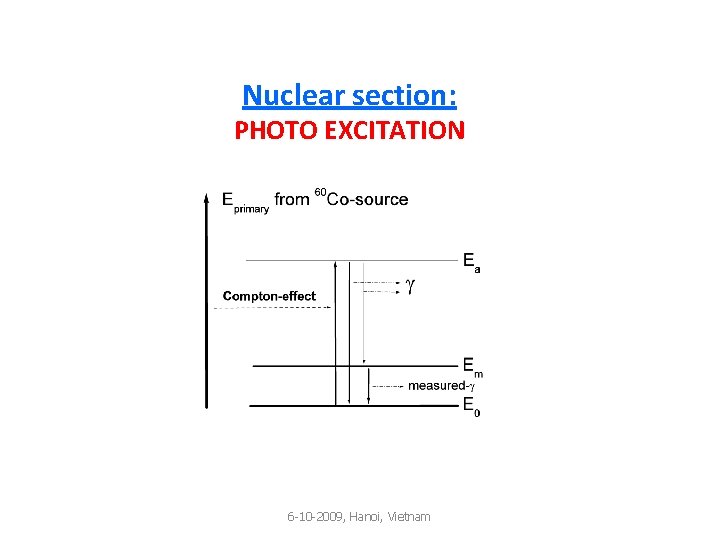
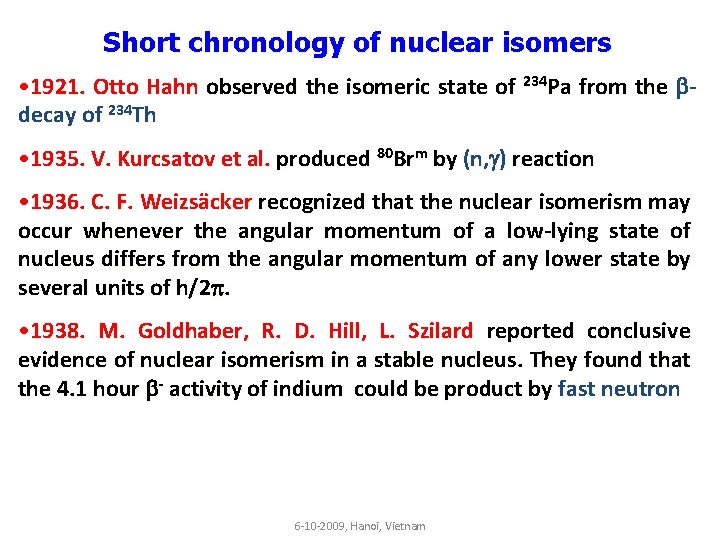
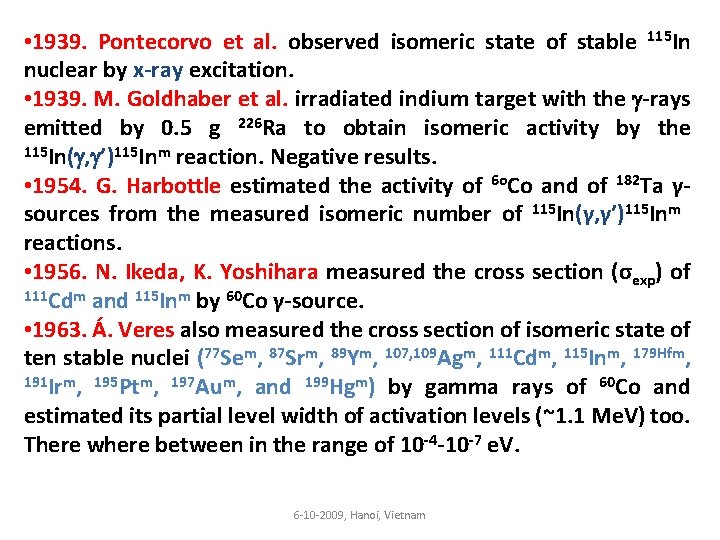
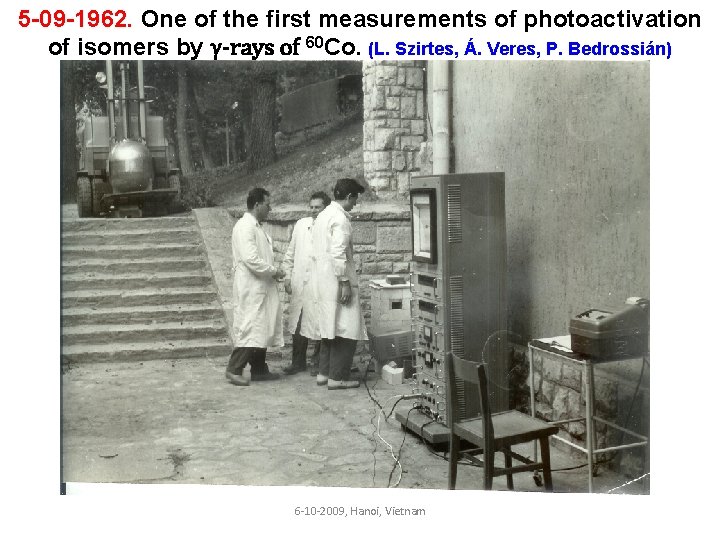
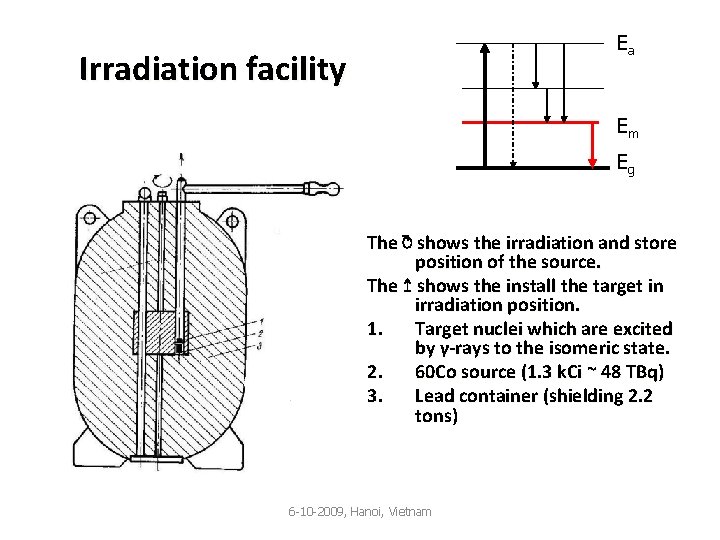
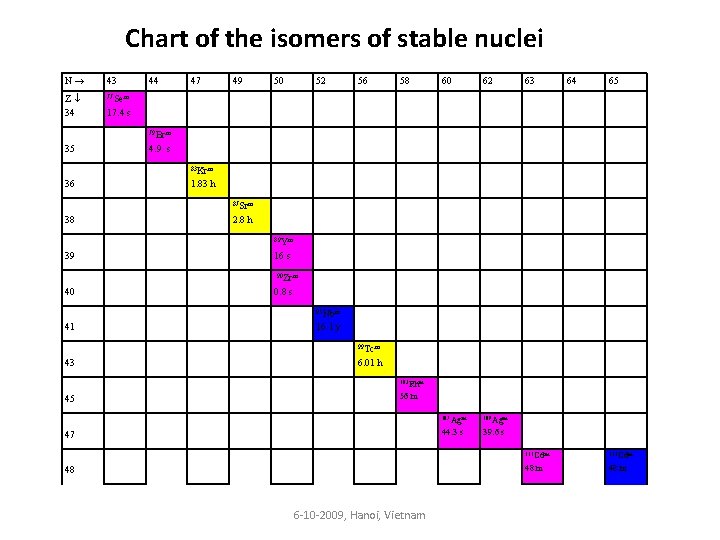
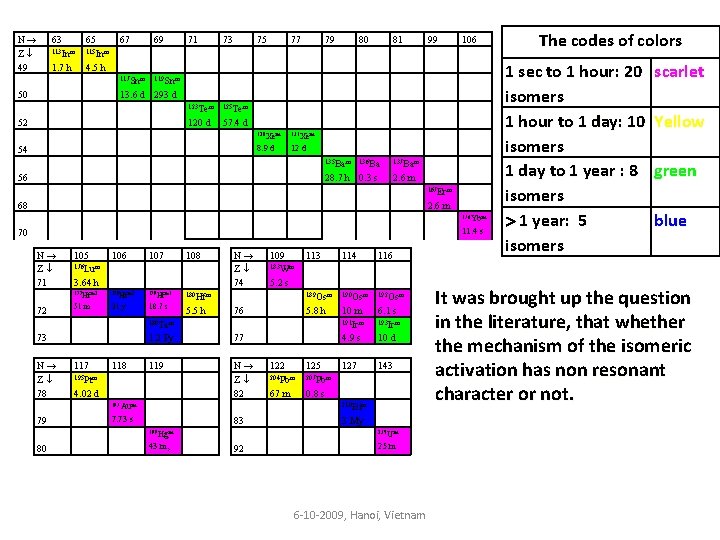
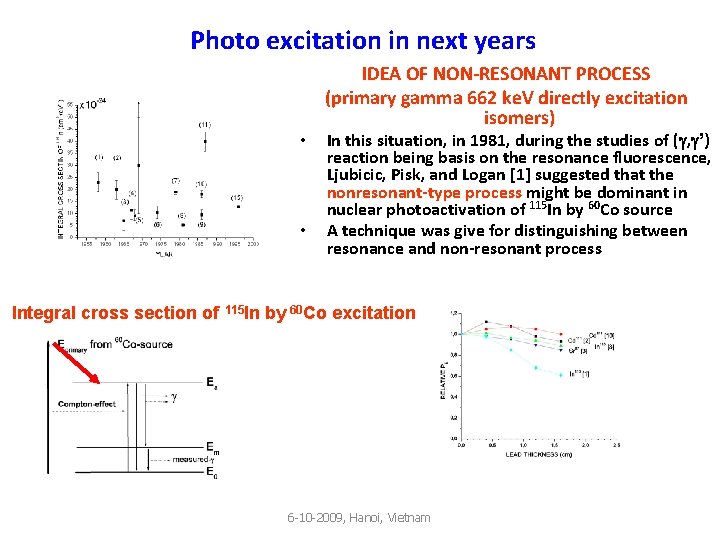
![NO-RESONANT and RESONANT [1] A. Ljubicic, K. Pisk, and B. A. Logan, Phys. Rev. NO-RESONANT and RESONANT [1] A. Ljubicic, K. Pisk, and B. A. Logan, Phys. Rev.](https://slidetodoc.com/presentation_image_h/26875a4587076570ba69672c75b11fd2/image-18.jpg)
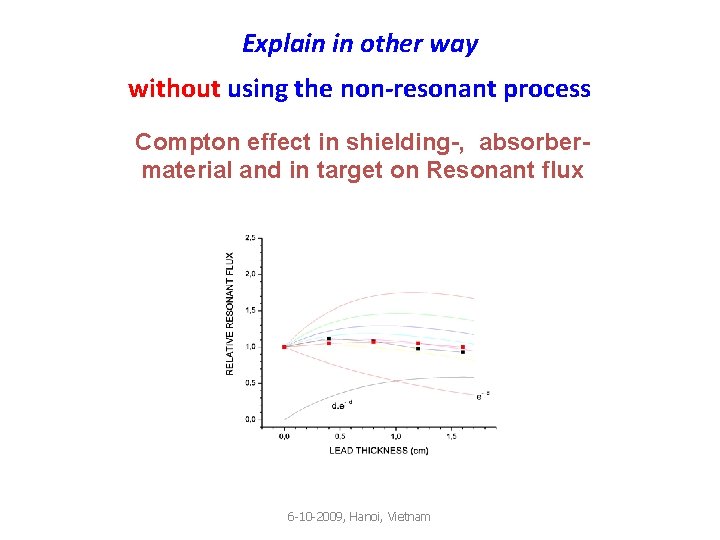
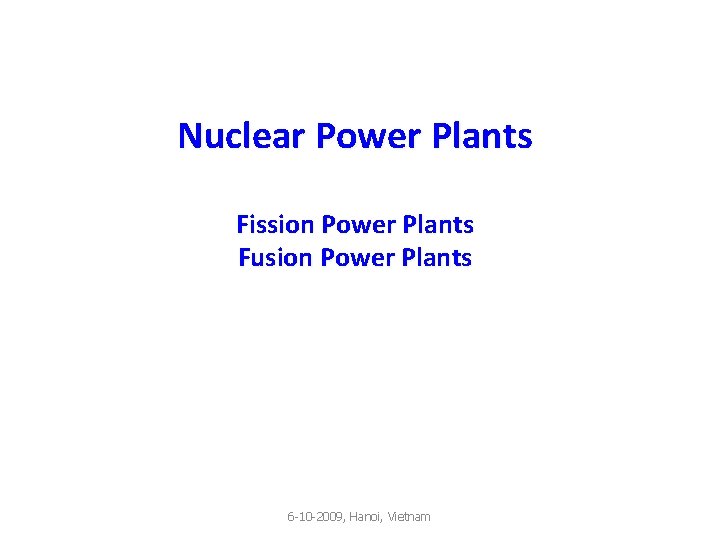
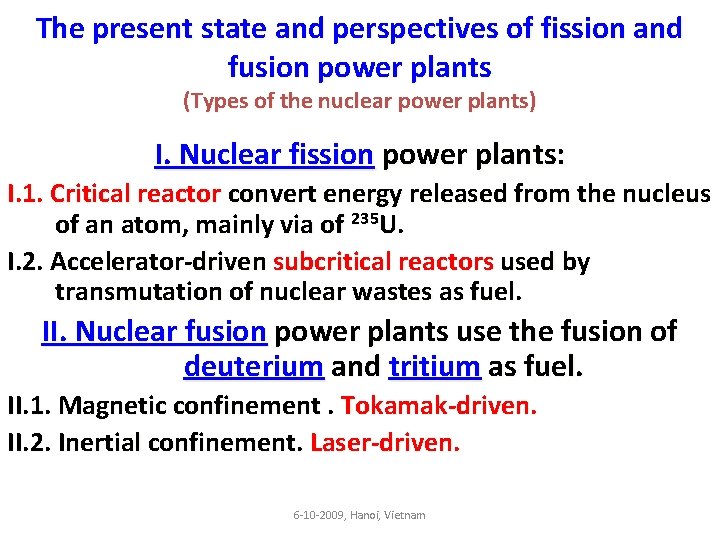
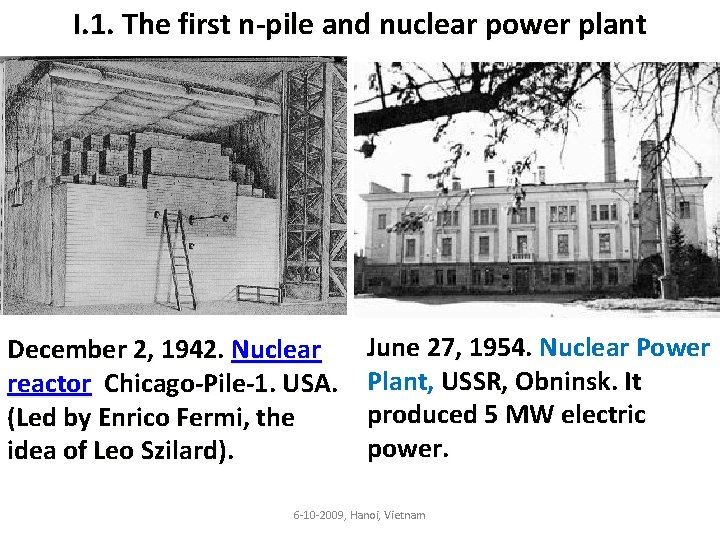
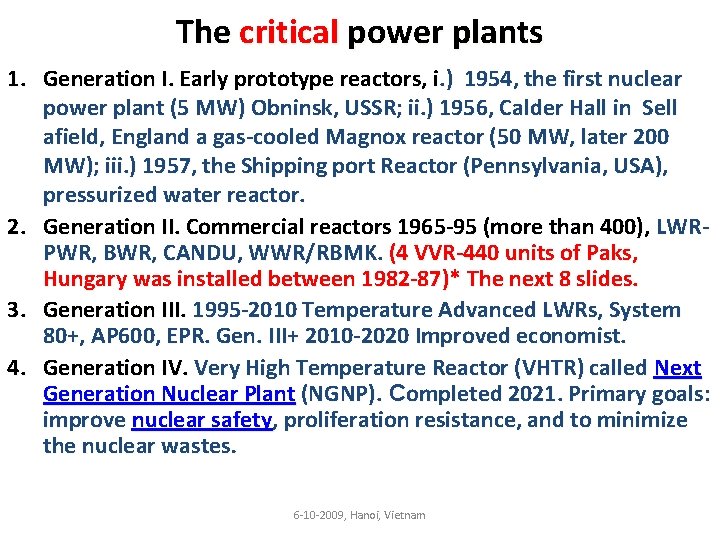
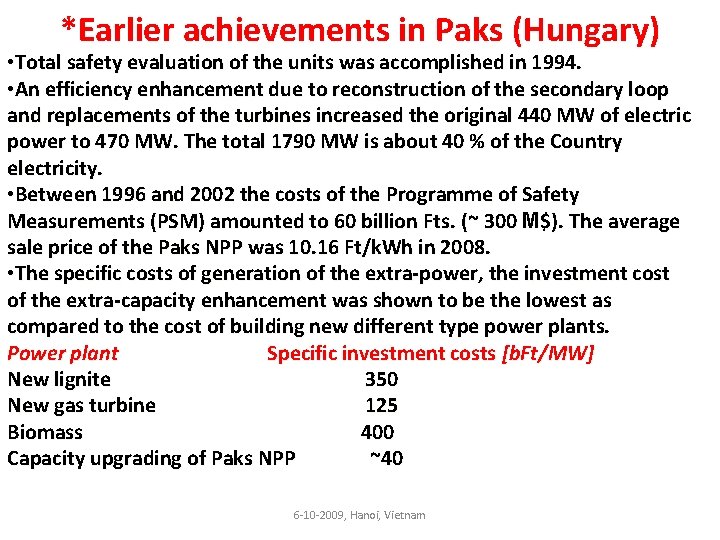
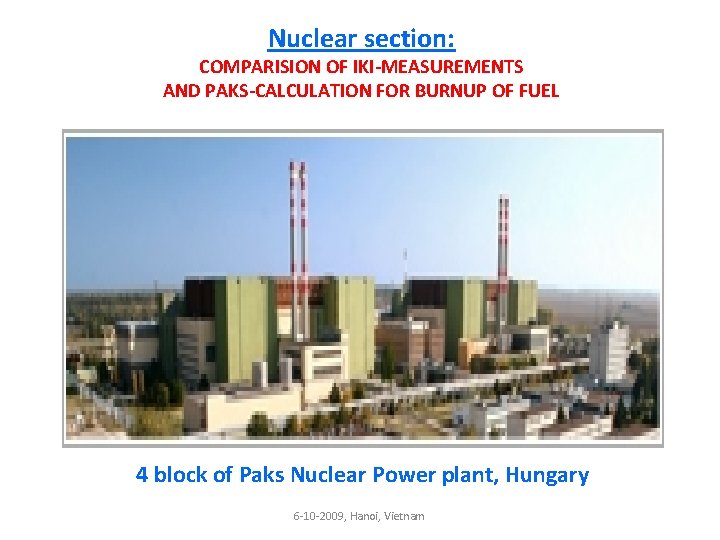
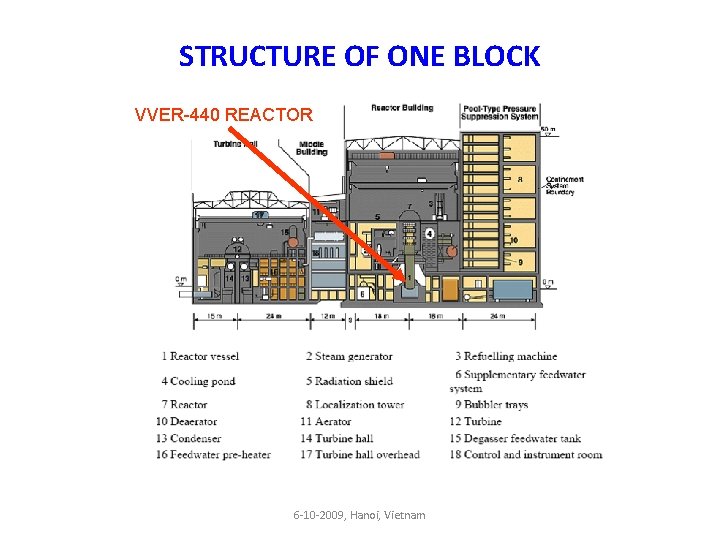
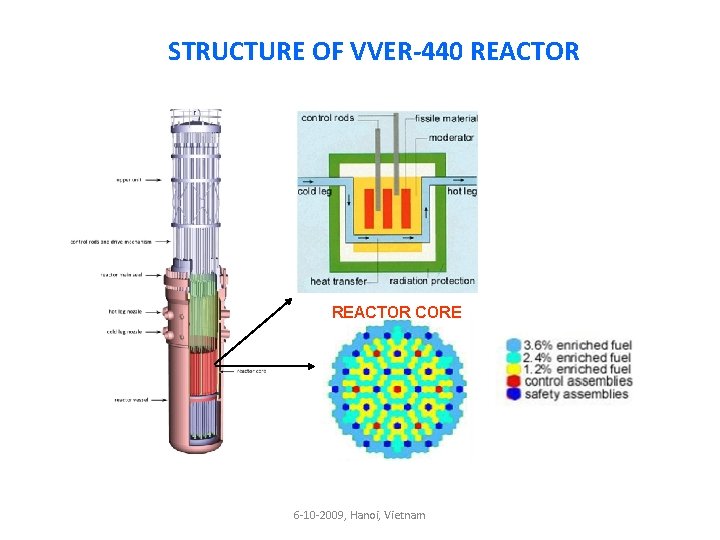
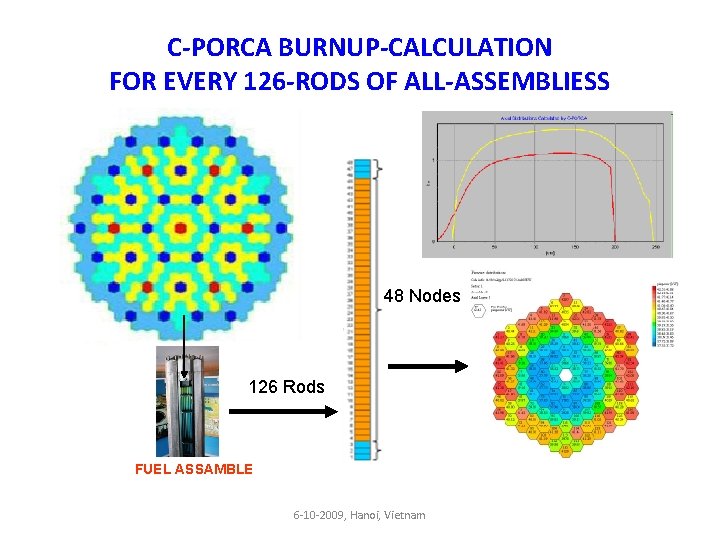
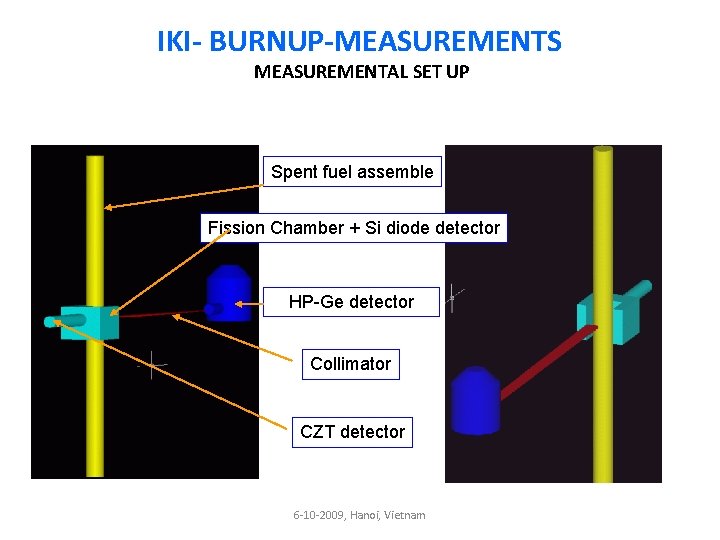
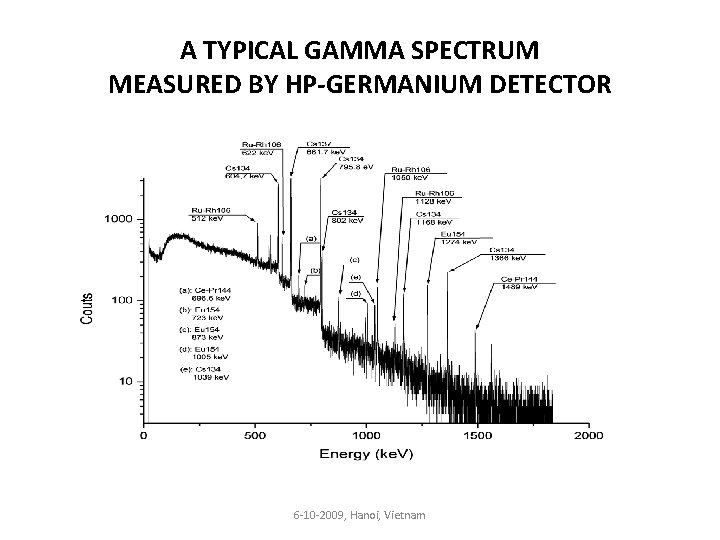
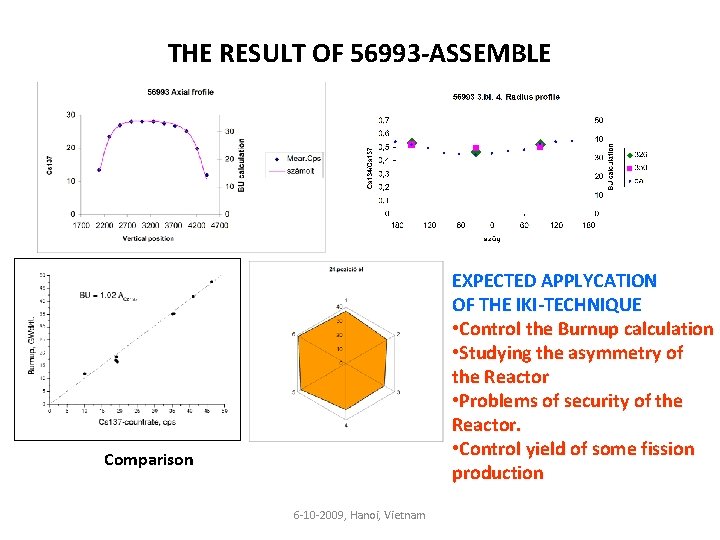
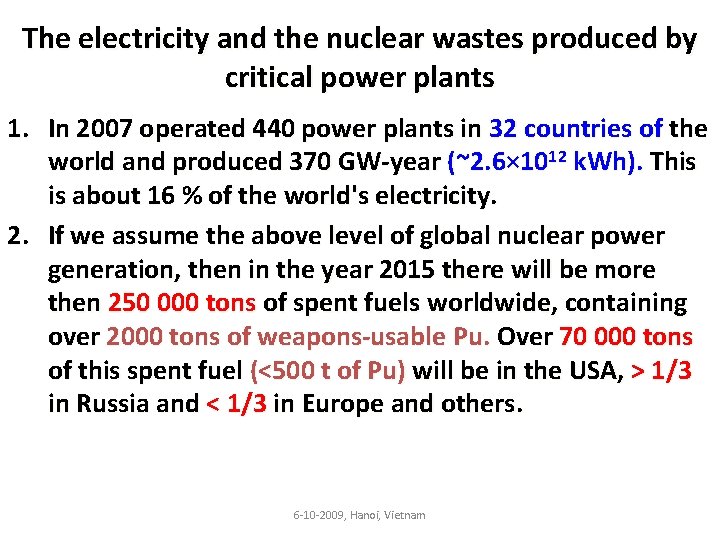
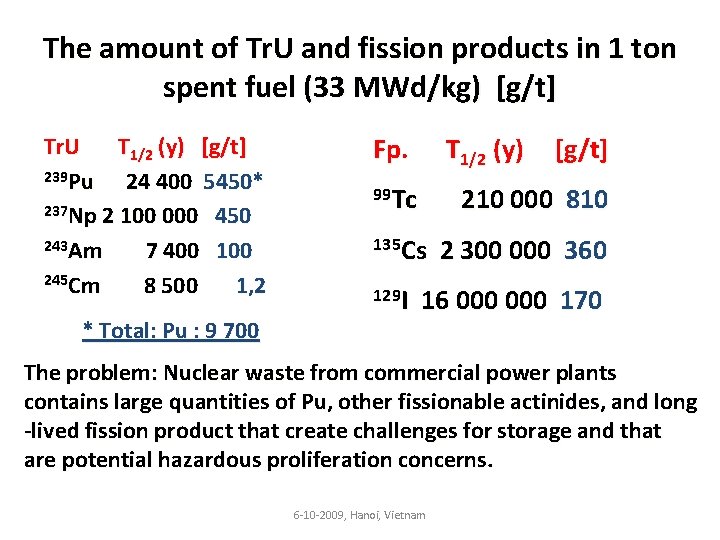
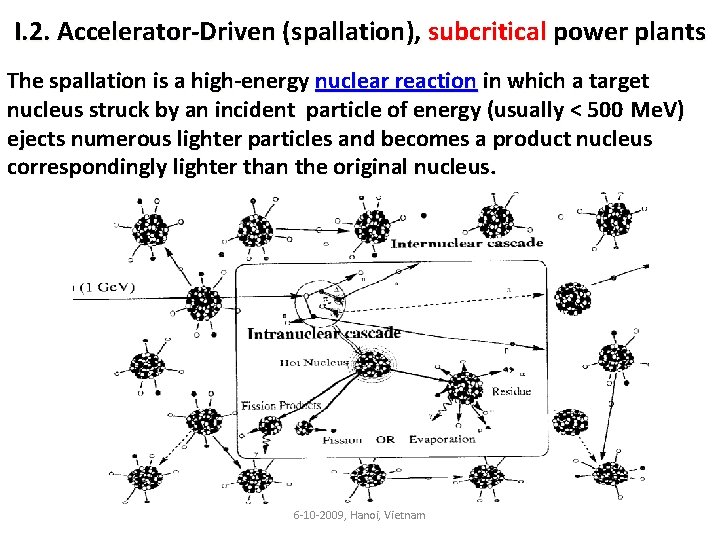
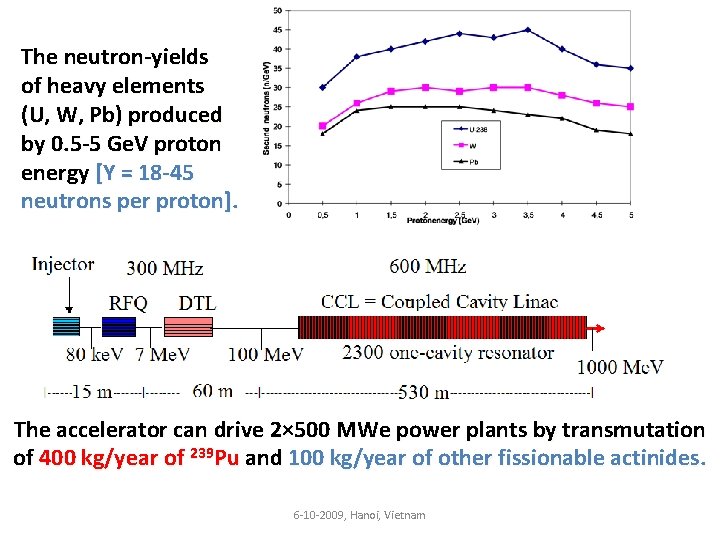
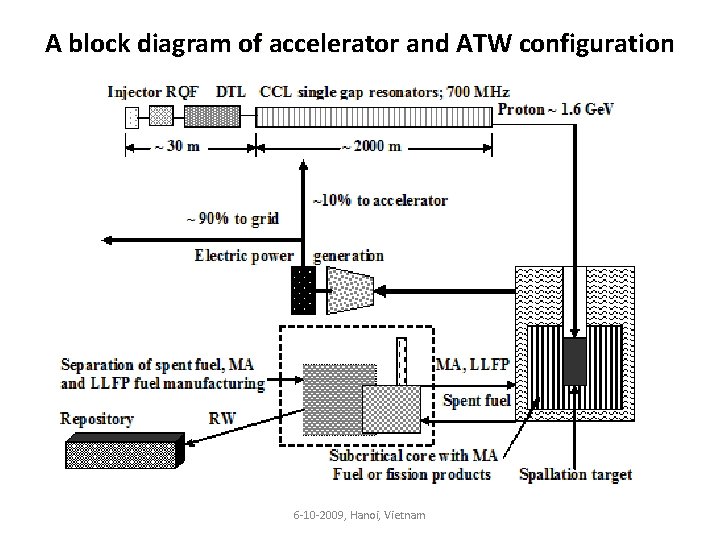
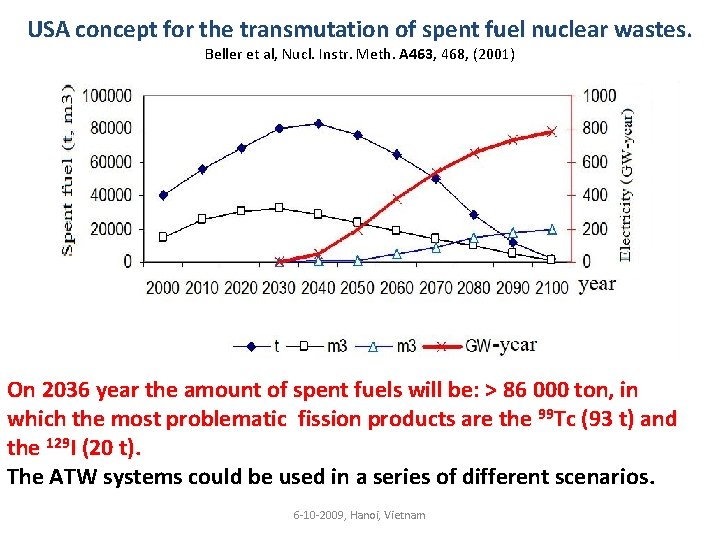
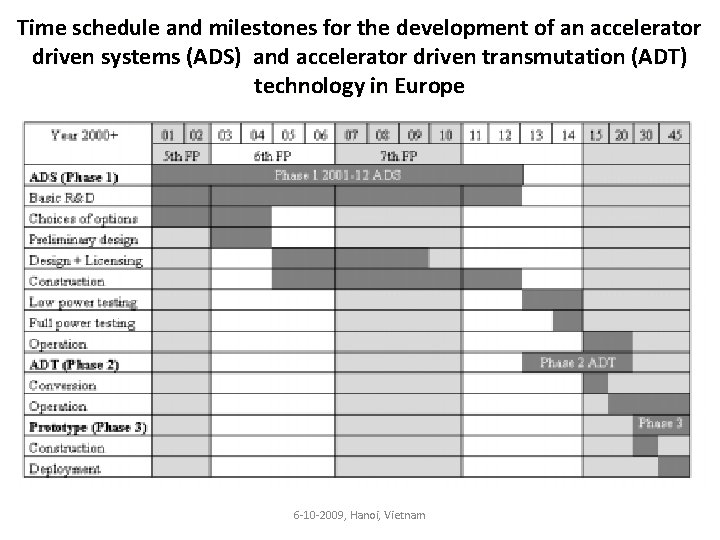
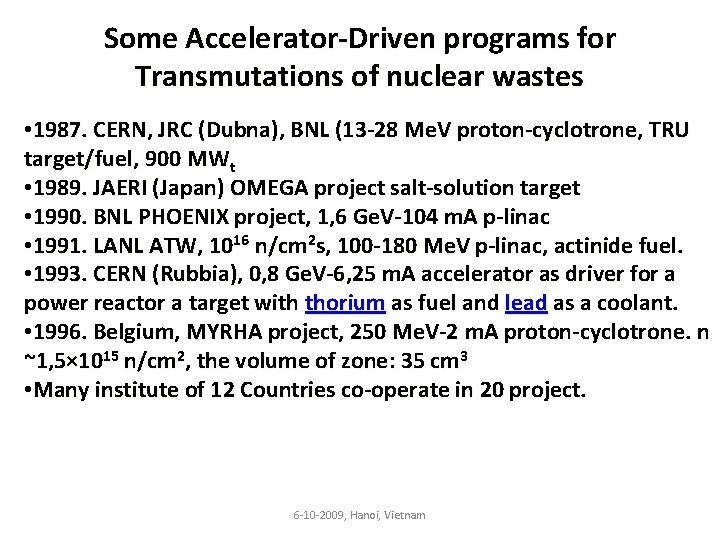
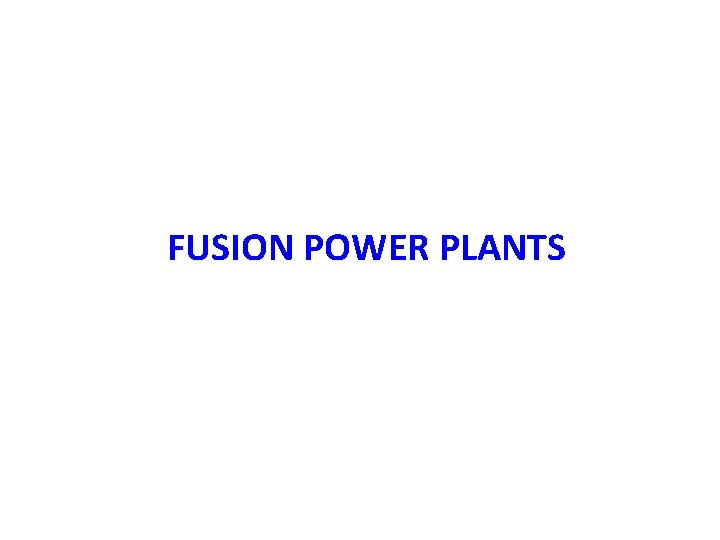
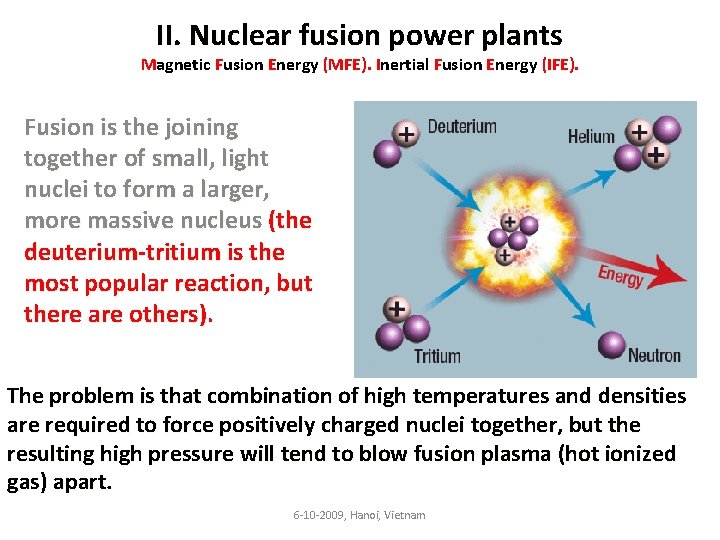
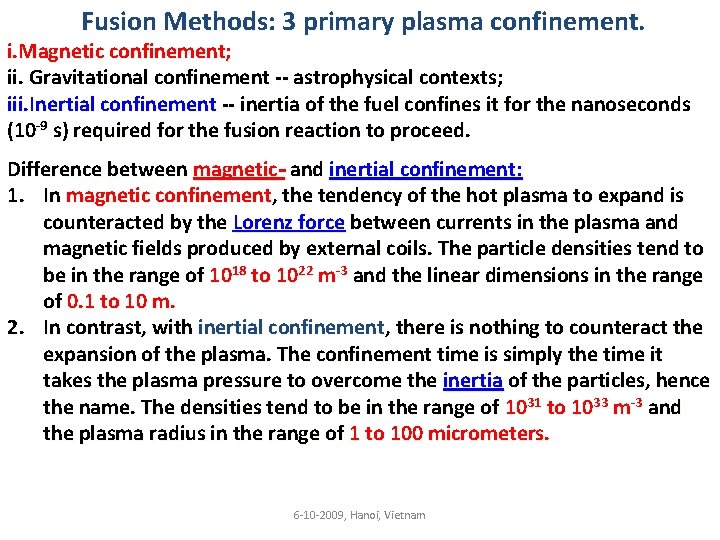
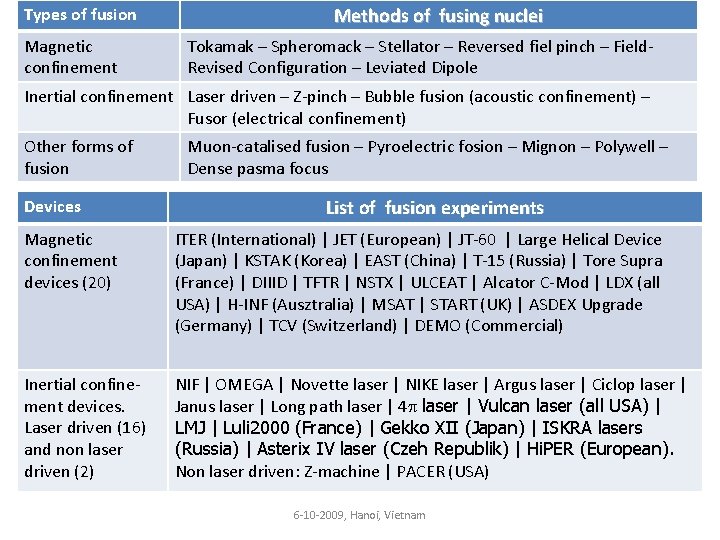
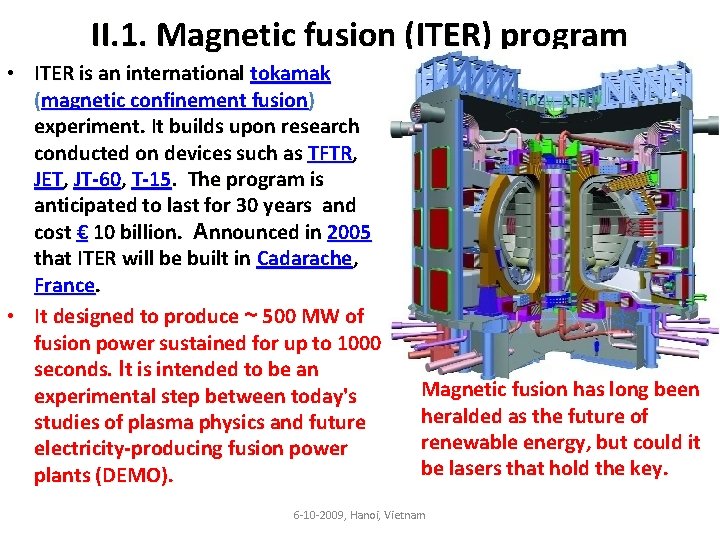
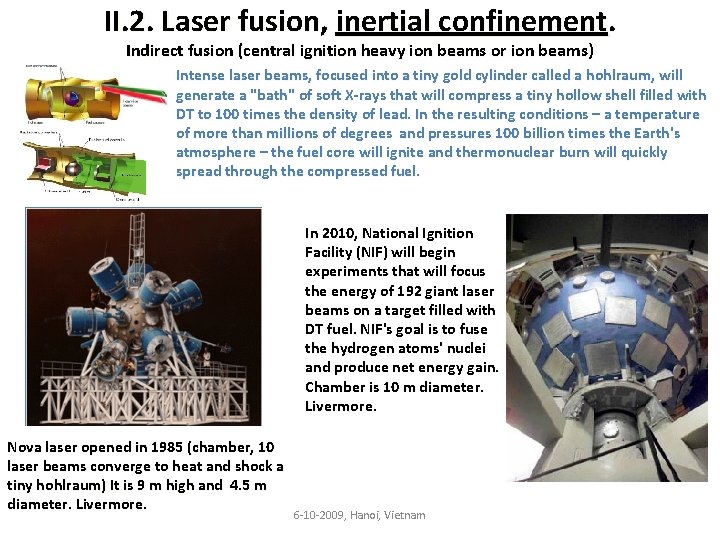
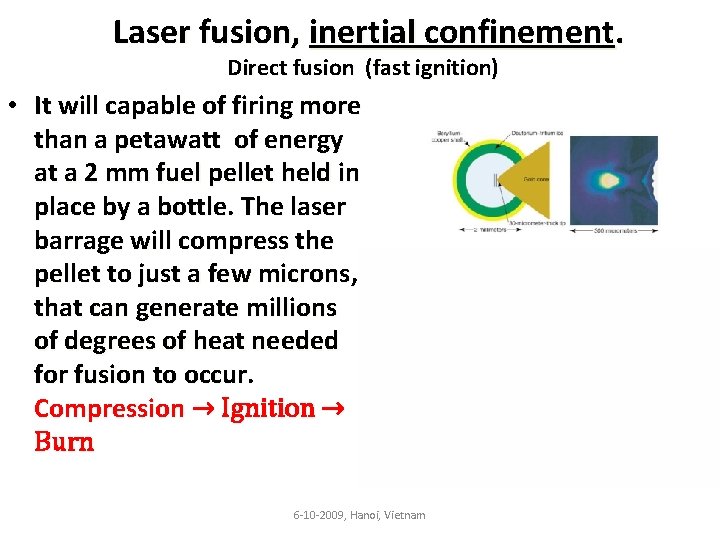
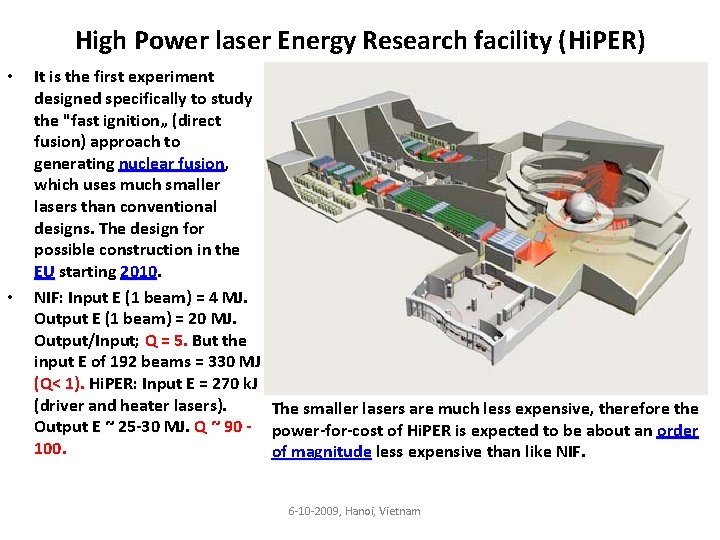
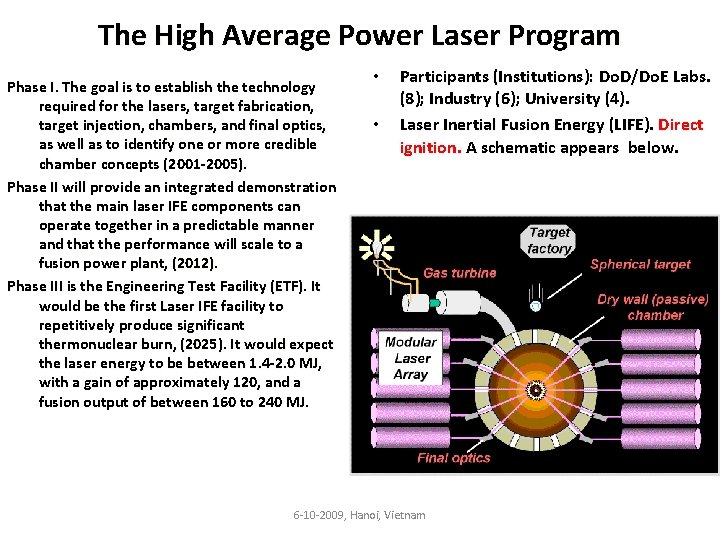
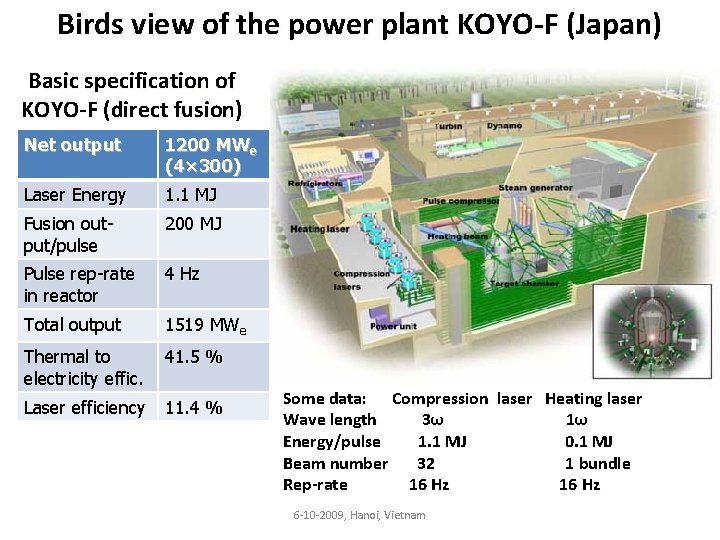
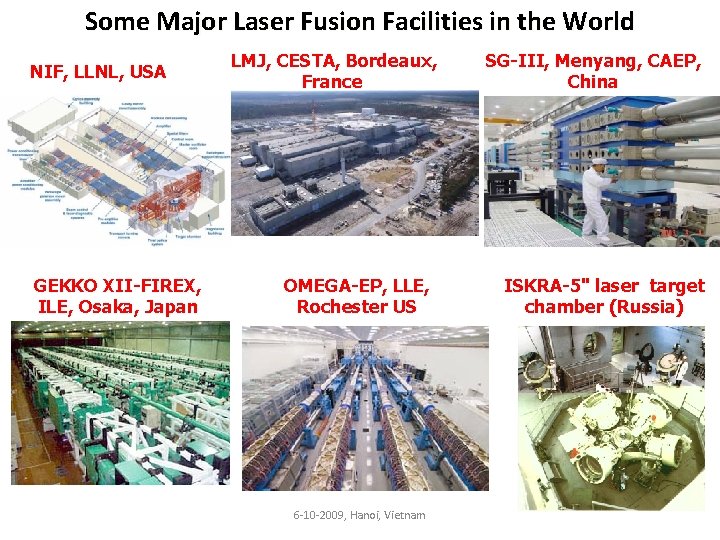
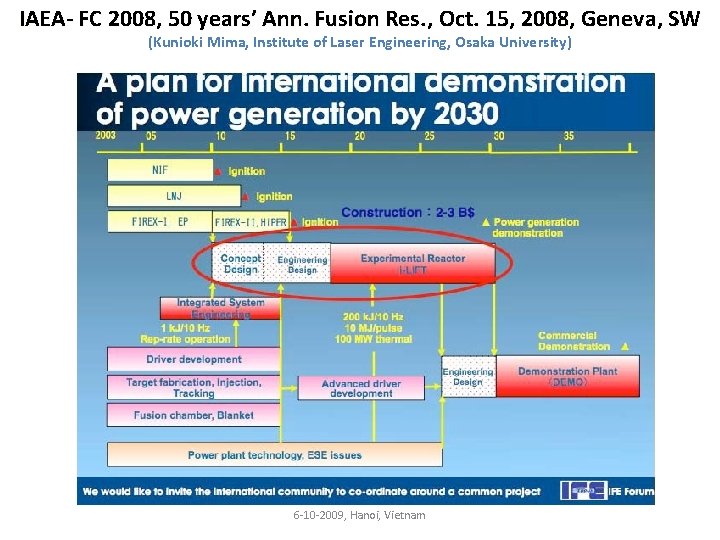
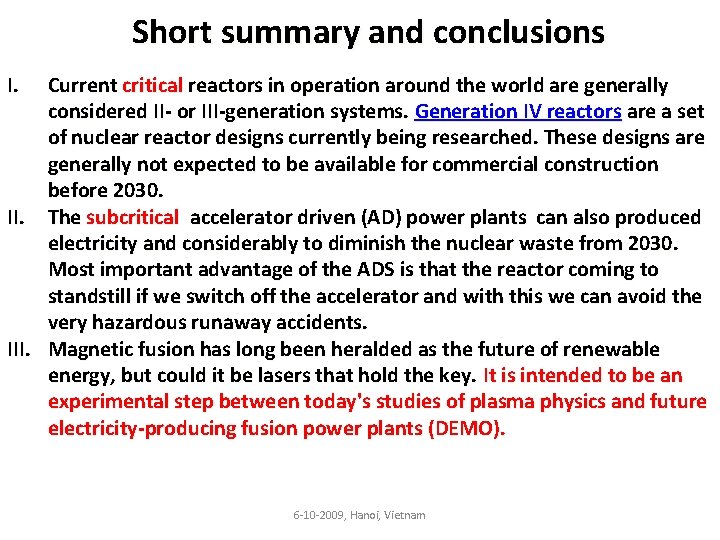
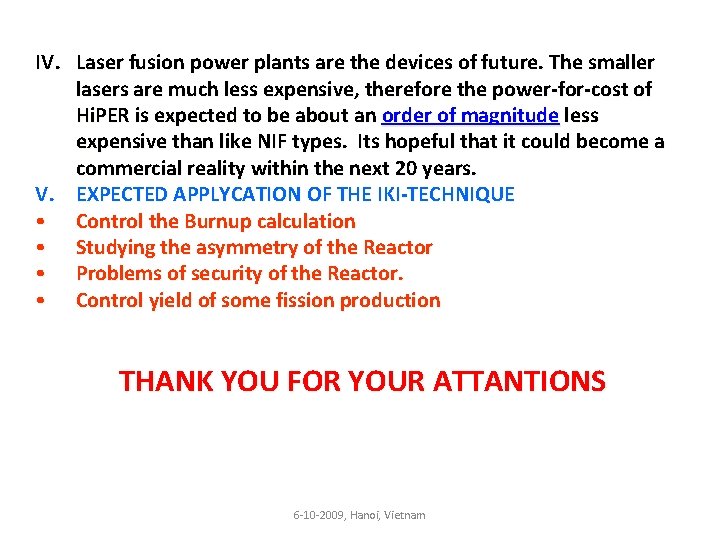
- Slides: 53
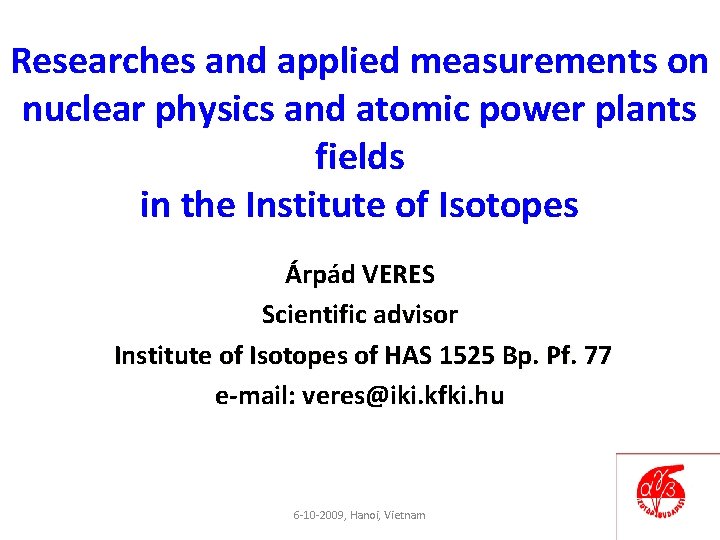
Researches and applied measurements on nuclear physics and atomic power plants fields in the Institute of Isotopes Árpád VERES Scientific advisor Institute of Isotopes of HAS 1525 Bp. Pf. 77 e-mail: veres@iki. kfki. hu 6 -10 -2009, Hanoi, Vietnam
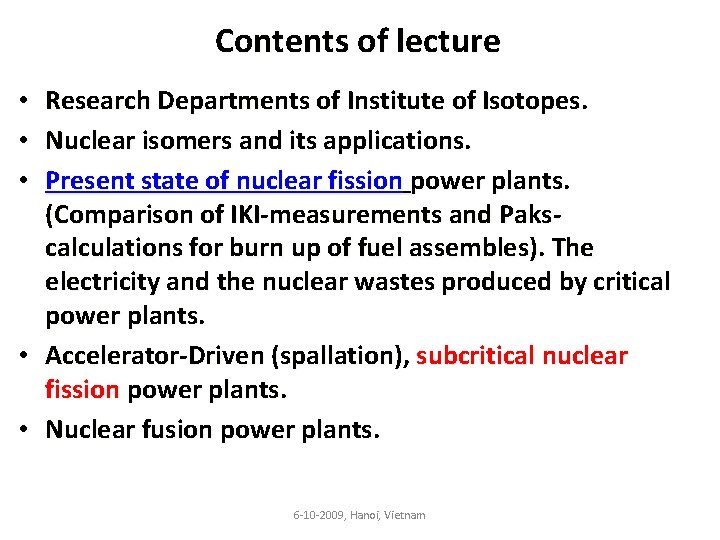
Contents of lecture • Research Departments of Institute of Isotopes. • Nuclear isomers and its applications. • Present state of nuclear fission power plants. (Comparison of IKI-measurements and Pakscalculations for burn up of fuel assembles). The electricity and the nuclear wastes produced by critical power plants. • Accelerator-Driven (spallation), subcritical nuclear fission power plants. • Nuclear fusion power plants. 6 -10 -2009, Hanoi, Vietnam
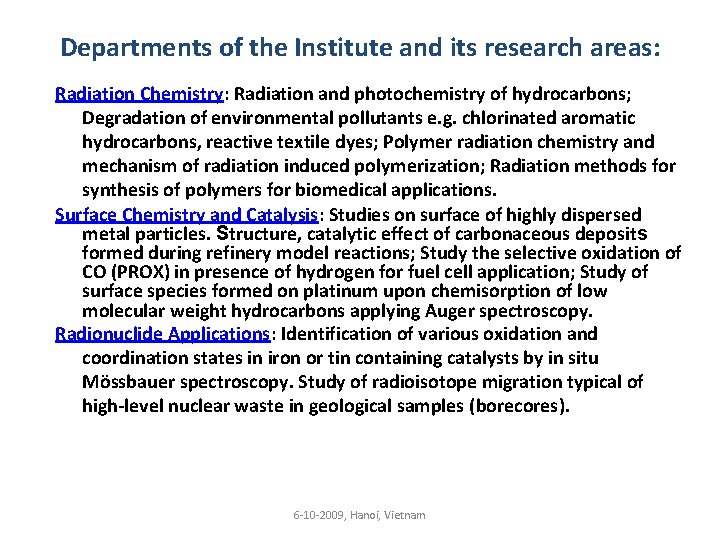
Departments of the Institute and its research areas: Radiation Chemistry: Radiation and photochemistry of hydrocarbons; Degradation of environmental pollutants e. g. chlorinated aromatic hydrocarbons, reactive textile dyes; Polymer radiation chemistry and mechanism of radiation induced polymerization; Radiation methods for synthesis of polymers for biomedical applications. Surface Chemistry and Catalysis: Studies on surface of highly dispersed metal particles. Structure, catalytic effect of carbonaceous deposits formed during refinery model reactions; Study the selective oxidation of CO (PROX) in presence of hydrogen for fuel cell application; Study of surface species formed on platinum upon chemisorption of low molecular weight hydrocarbons applying Auger spectroscopy. Radionuclide Applications: Identification of various oxidation and coordination states in iron or tin containing catalysts by in situ Mössbauer spectroscopy. Study of radioisotope migration typical of high-level nuclear waste in geological samples (borecores). 6 -10 -2009, Hanoi, Vietnam
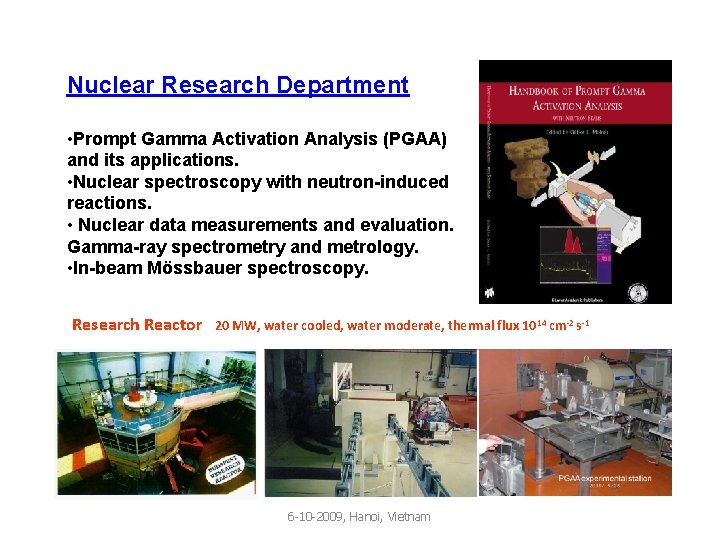
Nuclear Research Department • Prompt Gamma Activation Analysis (PGAA) and its applications. • Nuclear spectroscopy with neutron-induced reactions. • Nuclear data measurements and evaluation. Gamma-ray spectrometry and metrology. • In-beam Mössbauer spectroscopy. Research Reactor 20 MW, water cooled, water moderate, thermal flux 1014 cm-2 s-1 6 -10 -2009, Hanoi, Vietnam
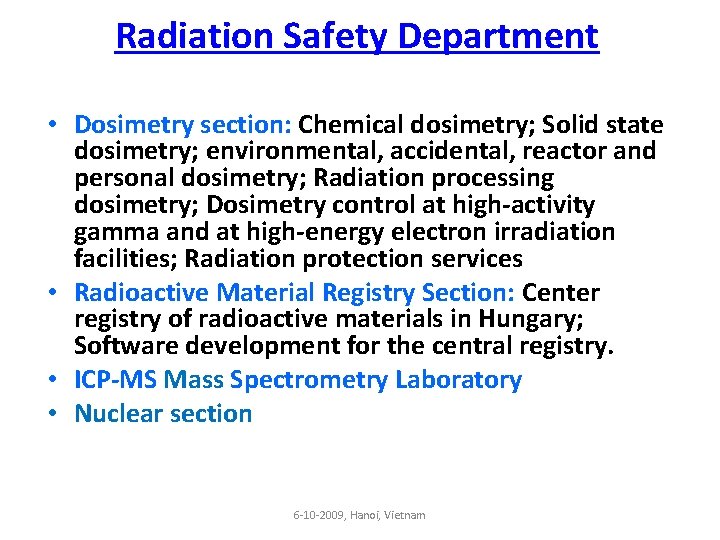
Radiation Safety Department • Dosimetry section: Chemical dosimetry; Solid state dosimetry; environmental, accidental, reactor and personal dosimetry; Radiation processing dosimetry; Dosimetry control at high-activity gamma and at high-energy electron irradiation facilities; Radiation protection services • Radioactive Material Registry Section: Center registry of radioactive materials in Hungary; Software development for the central registry. • ICP-MS Mass Spectrometry Laboratory • Nuclear section 6 -10 -2009, Hanoi, Vietnam
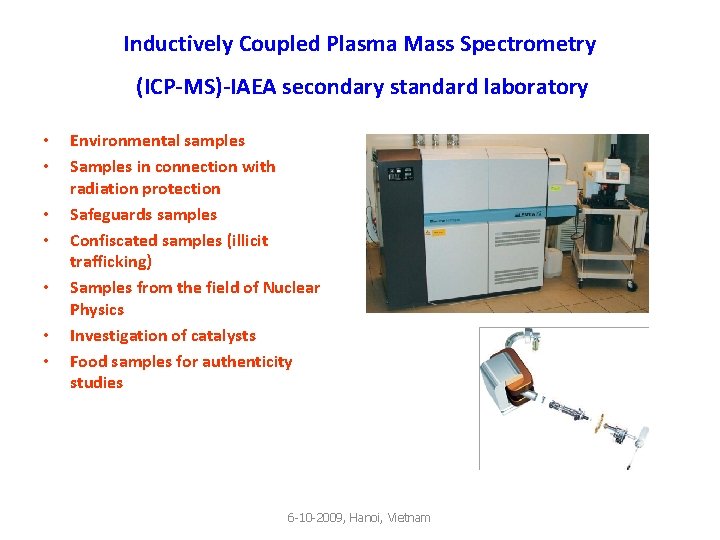
Inductively Coupled Plasma Mass Spectrometry (ICP-MS)-IAEA secondary standard laboratory • • Environmental samples Samples in connection with radiation protection Safeguards samples Confiscated samples (illicit trafficking) Samples from the field of Nuclear Physics Investigation of catalysts Food samples for authenticity studies 6 -10 -2009, Hanoi, Vietnam
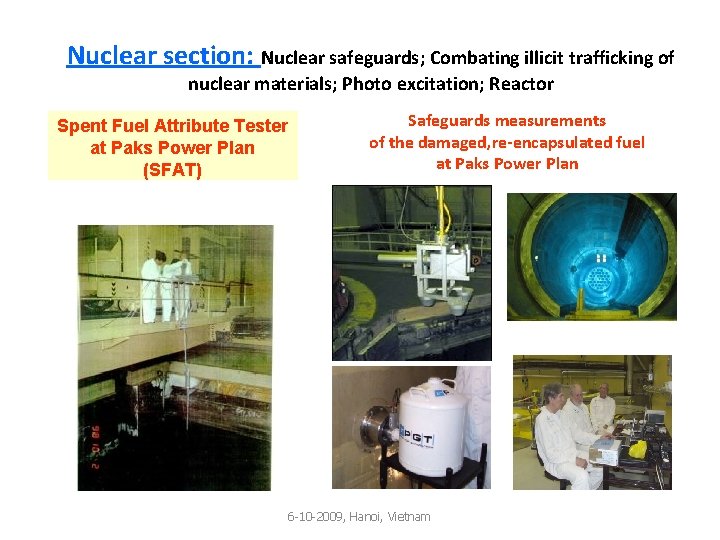
Nuclear section: Nuclear safeguards; Combating illicit trafficking of nuclear materials; Photo excitation; Reactor Spent Fuel Attribute Tester at Paks Power Plan (SFAT) Safeguards measurements of the damaged, re-encapsulated fuel at Paks Power Plan 6 -10 -2009, Hanoi, Vietnam
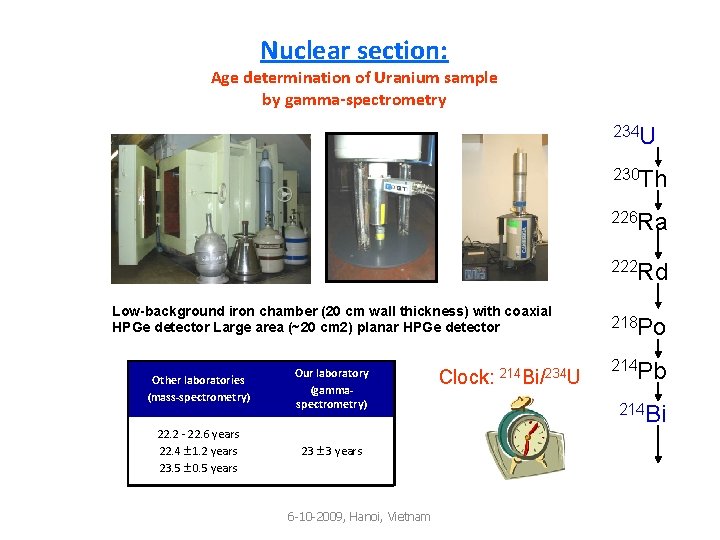
Nuclear section: Age determination of Uranium sample by gamma-spectrometry 234 U 230 Th 226 Ra 222 Rd Low-background iron chamber (20 cm wall thickness) with coaxial HPGe detector Large area (~20 cm 2) planar HPGe detector Other laboratories (mass-spectrometry) Our laboratory (gammaspectrometry) 22. 2 - 22. 6 years 22. 4 1. 2 years 23. 5 0. 5 years 23 3 years 6 -10 -2009, Hanoi, Vietnam Clock: 214 Bi/234 U 218 Po 214 Pb 214 Bi
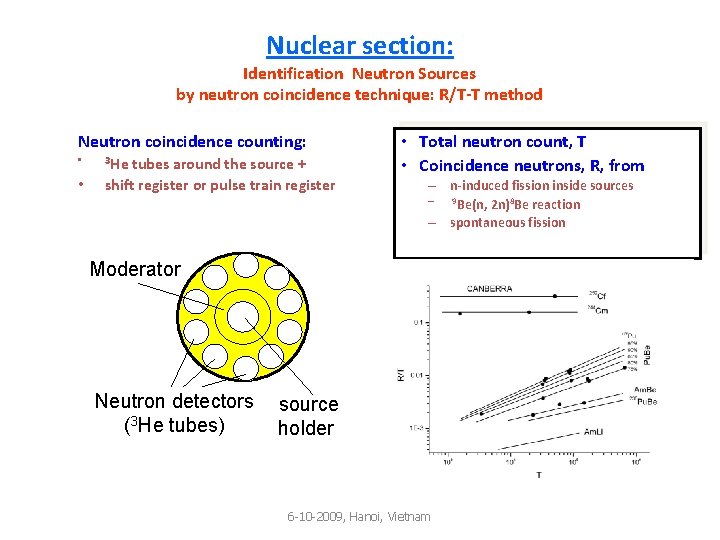
Nuclear section: Identification Neutron Sources by neutron coincidence technique: R/T-T method Neutron coincidence counting: • 3 He tubes around the source + • shift register or pulse train register • Total neutron count, T • Coincidence neutrons, R, from – n-induced fission inside sources – 9 Be(n, 2 n)8 Be reaction – spontaneous fission Moderator Neutron detectors (3 He tubes) source holder 6 -10 -2009, Hanoi, Vietnam
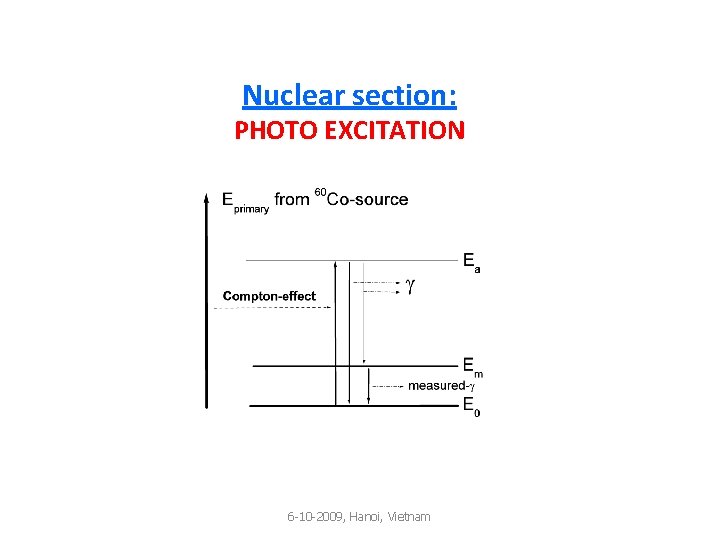
Nuclear section: PHOTO EXCITATION 6 -10 -2009, Hanoi, Vietnam
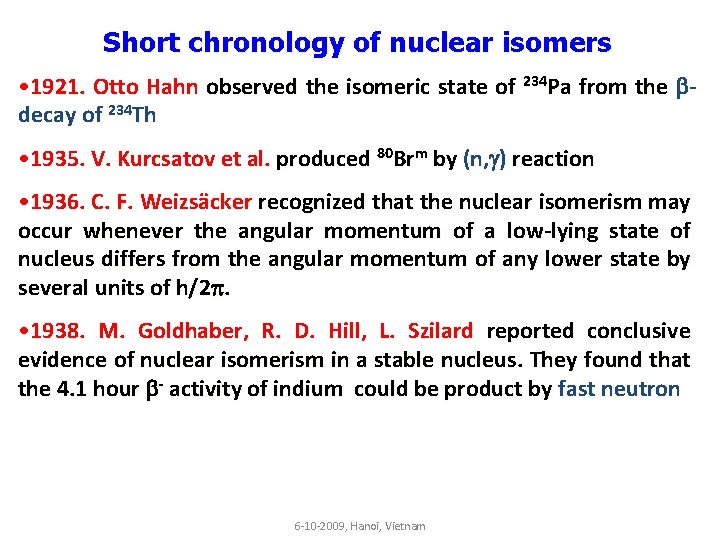
Short chronology of nuclear isomers • 1921. Otto Hahn observed the isomeric state of 234 Pa from the decay of 234 Th • 1935. V. Kurcsatov et al. produced 80 Brm by (n, ) reaction • 1936. C. F. Weizsäcker recognized that the nuclear isomerism may occur whenever the angular momentum of a low-lying state of nucleus differs from the angular momentum of any lower state by several units of h/2. • 1938. M. Goldhaber, R. D. Hill, L. Szilard reported conclusive evidence of nuclear isomerism in a stable nucleus. They found that the 4. 1 hour - activity of indium could be product by fast neutron 6 -10 -2009, Hanoi, Vietnam
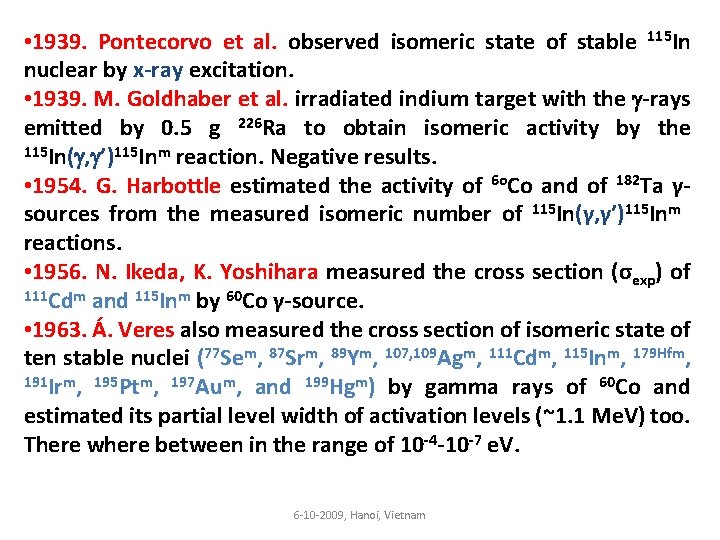
• 1939. Pontecorvo et al. observed isomeric state of stable 115 In nuclear by x-ray excitation. • 1939. M. Goldhaber et al. irradiated indium target with the -rays emitted by 0. 5 g 226 Ra to obtain isomeric activity by the 115 In( , ’)115 Inm reaction. Negative results. • 1954. G. Harbottle estimated the activity of 6 o. Co and of 182 Ta γ- sources from the measured isomeric number of 115 In(γ, γ’)115 Inm reactions. • 1956. N. Ikeda, K. Yoshihara measured the cross section (σexp) of 111 Cdm and 115 Inm by 60 Co γ-source. • 1963. Á. Veres also measured the cross section of isomeric state of ten stable nuclei (77 Sem, 87 Srm, 89 Ym, 107, 109 Agm, 111 Cdm, 115 Inm, 179 Hfm, 191 Irm, 195 Ptm, 197 Aum, and 199 Hgm) by gamma rays of 60 Co and estimated its partial level width of activation levels (~1. 1 Me. V) too. There where between in the range of 10 -4 -10 -7 e. V. 6 -10 -2009, Hanoi, Vietnam
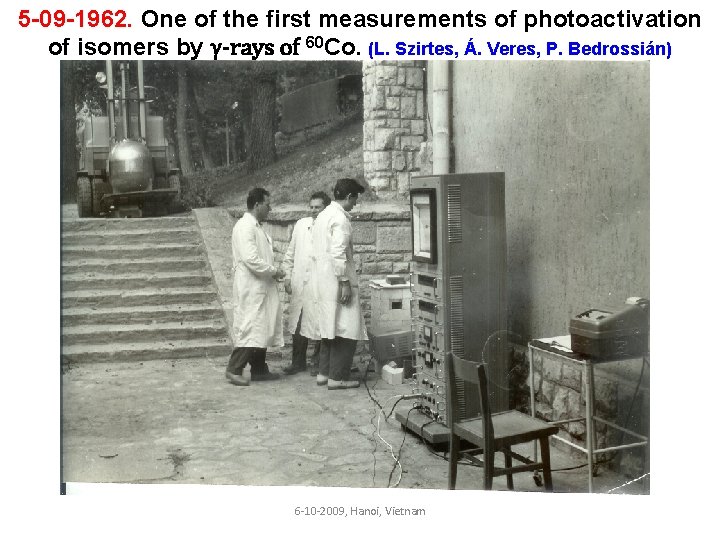
5 -09 -1962. One of the first measurements of photoactivation of isomers by γ-rays of 60 Co. (L. Szirtes, Á. Veres, P. Bedrossián) 6 -10 -2009, Hanoi, Vietnam
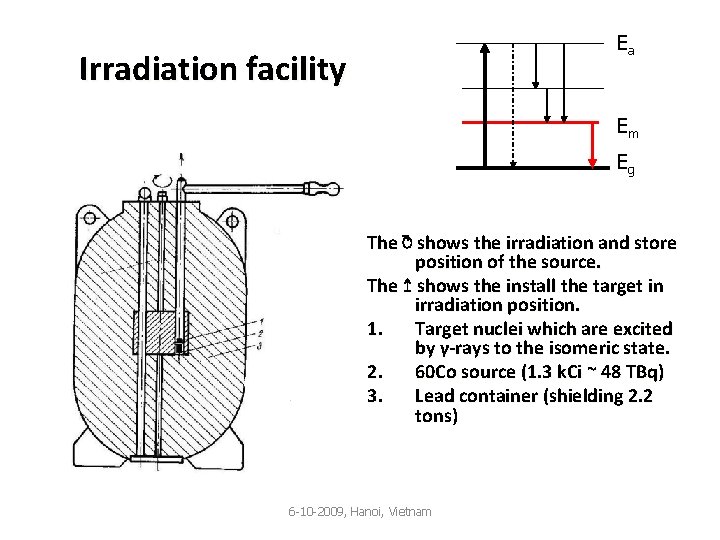
Ea Irradiation facility Em Eg The ↻ shows the irradiation and store position of the source. The ↥ shows the install the target in irradiation position. 1. Target nuclei which are excited by γ-rays to the isomeric state. 2. 60 Co source (1. 3 k. Ci ~ 48 TBq) 3. Lead container (shielding 2. 2 tons) 6 -10 -2009, Hanoi, Vietnam
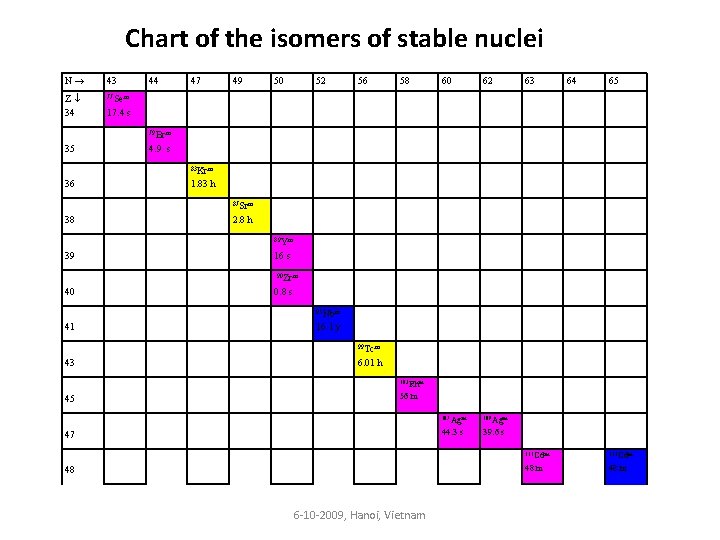
Chart of the isomers of stable nuclei N 43 Z 34 77 Sem 44 47 49 50 52 56 58 60 62 107 Agm 109 Agm 44. 3 s 39. 6 s 63 64 65 17. 4 s 79 Brm 35 4. 9 s 83 Krm 36 1. 83 h 87 Srm 38 2. 8 h 89 Ym 39 16 s 90 Zrm 40 0. 8 s 93 Nbm 41 16. 1 y 99 Tcm 43 6. 01 h 103 Rhm 45 56 m 47 48 6 -10 -2009, Hanoi, Vietnam 111 Cdm 48 m
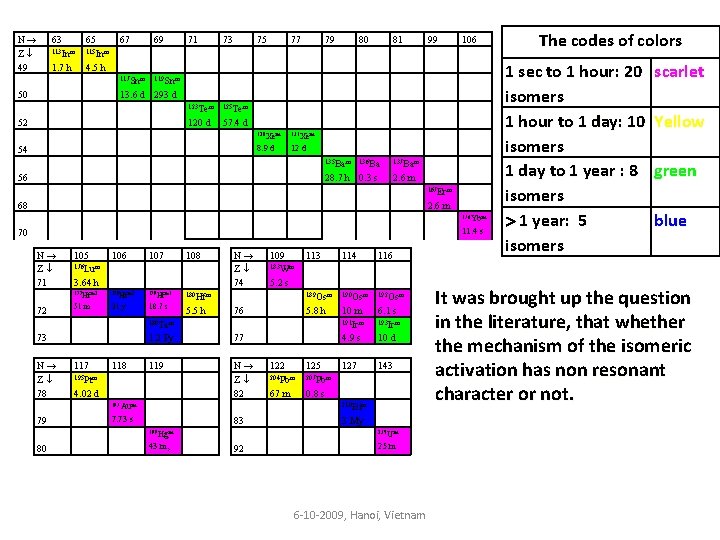
N Z 49 63 113 Inm 1. 7 h 65 115 Inm 4. 5 h 50 67 69 117 Snm 119 Snm 71 73 75 77 79 80 81 99 106 13. 6 d 293 d 52 123 Tem 125 Tem 120 d 57. 4 d 54 120 Xem 131 Xem 8. 9 d 12 d 135 Bam 56 136 Ba 28. 7 h 0. 3 s 137 Bam 2. 6 m 167 Erm 68 2. 6 m 176 Ybm 11. 4 s 70 N Z 71 72 105 176 Lum 3. 64 h 106 107 108 177 Hfm 2 178 Hfm 2 179 Hfm 1 51 m 31 y 18. 7 s 180 Hfm 5. 5 h N Z 74 109 183 Wm 5. 2 s 76 113 114 116 189 Osm 190 Osm 192 Osm 5. 8 h 10 m 191 Irm 4. 9 s 6. 1 s 193 Irm 10 d 125 207 Pbm 0. 8 s 127 143 180 Tam 73 N Z 78 117 195 Ptm 4. 02 d 118 1. 2 Py 77 119 N Z 82 197 Aum 79 210 Bim 7. 73 s 83 199 Hgm 80 122 204 Pbm 67 m 43 m, 3 My 235 Um 92 25 m 6 -10 -2009, Hanoi, Vietnam The codes of colors 1 sec to 1 hour: 20 scarlet isomers 1 hour to 1 day: 10 Yellow isomers 1 day to 1 year : 8 green isomers 1 year: 5 blue isomers It was brought up the question in the literature, that whether the mechanism of the isomeric activation has non resonant character or not.
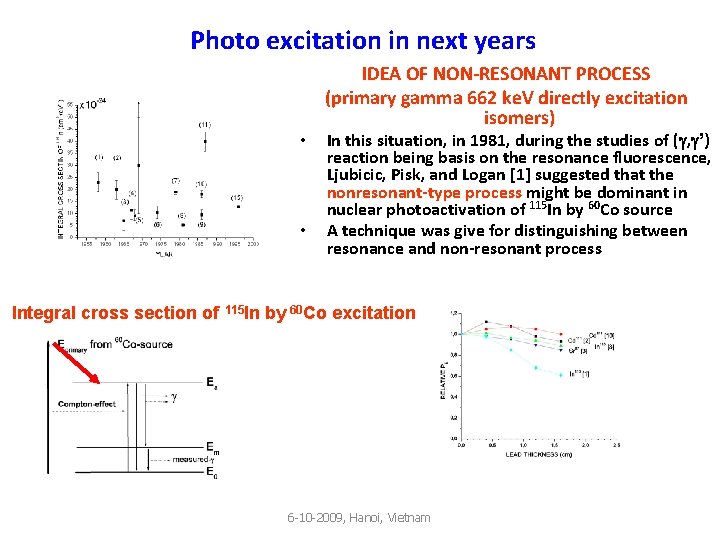
Photo excitation in next years • • IDEA OF NON-RESONANT PROCESS (primary gamma 662 ke. V directly excitation isomers) In this situation, in 1981, during the studies of ( , ’) reaction being basis on the resonance fluorescence, Ljubicic, Pisk, and Logan [1] suggested that the nonresonant-type process might be dominant in nuclear photoactivation of 115 In by 60 Co source A technique was give for distinguishing between resonance and non-resonant process Integral cross section of 115 In by 60 Co excitation 6 -10 -2009, Hanoi, Vietnam
![NORESONANT and RESONANT 1 A Ljubicic K Pisk and B A Logan Phys Rev NO-RESONANT and RESONANT [1] A. Ljubicic, K. Pisk, and B. A. Logan, Phys. Rev.](https://slidetodoc.com/presentation_image_h/26875a4587076570ba69672c75b11fd2/image-18.jpg)
NO-RESONANT and RESONANT [1] A. Ljubicic, K. Pisk, and B. A. Logan, Phys. Rev. C 23, 2238 (1981). [2] M. Krcmar, A. Ljubicic, K. Pisk, B. A. Logan, and M. Vrtar, Phys. Rev. C 25, 2097 (1982). [3] M. Krcmar, A. Ljubicic, B. A. Logan, and M. Bistrovic, Phys. Rev. C 33, 293 (1986). [4] K. Yoshihara, Zs. Nemeth, L. Lakosi, I. Pavlicsek, and A. Veres, Phys. Rev. C 33, 728 (1986). [5]I. Bikit, J. Slivka, I. Anicin, L. Marinkov, A. Rudic, and W. D. Hamilton, Universitet Novi Sad Report 17. Physics Seriet (1987). [6] I. Bikit, J. Slivka, I. Anicin, L. Marinkov, A. Rudic, and W. D. Hamilton, Phys. Rev. C 35, 1943 (1987). [7] J. A. Anderson, M. J. Byrd, and C. B. Collins, Phys. Rev. C 38, 2838 (1988). [8] P. von. Neumann-Cosel, A. Richter, J. J. Carroll and C. B. Collins Phys. Rev. C 14, 554 (1991). [9] M. Krcmar, S. Cancic, T. Tustonic, A. Ljubicic, B. A. Logan, and M. Bistrovic, Phys. Rev. C 41, 771 (1990). [10] M. Krcmar, A. Ljubicic, B. A. Logan, and M. Bistrovic, Phys. Rev. C 47, 906 (1993). [11] T. Tustonic, ] M. Krcmar, A. Ljubicic and M. Bistrovic, Appl. Radiat. Isot. 48, 45 (1997). [12] D. A. Bradby, Ithnin Abdul Jalil, M. Krcmar, and A. Ljubicic, Journal of Radianalytical and Nuclear Chemistry 244, 475 (2000). [13] Chea-Beng Lee, D. A. Bradley, Ithnin Abdul Jalil, Y. M. Amin, Mohd Jamil Maah, Khairul Zaman M. Dahlan, Radiat. Phys. Chem. 61, 367 (2001). [14] K. Pisk, M. Krcmar, A. Ljubicic, and B. A. Logan, Phys. Rev. C 25, 2226 (1982). [15] M. Krcmar, A. Ljubicic, and K. Pisk, FZKAAA 18, 171 (1986). [16] Ljubicic, Radiat. Phys. Chem. 51, 341 (1998). [17] Drukarev. 2000. No-resonant excitation of nuclear levels by photon. In: R. W. Dunford, D. S. Gemmel, E. B. Kanter, B. Krassig, S. H. Southworth, L. Young, (Eds), X-rays and Inner Sell Processes, 18 th International Conference, Chicago, IL, August, AIP Conference Proceedings 506, American Institute of Physics, Melville, NY, pp. 496 -500 6 -10 -2009, Hanoi, Vietnam
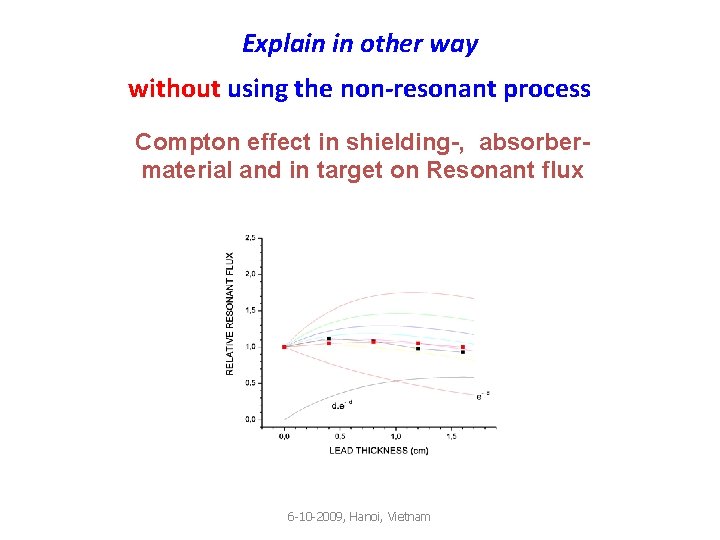
Explain in other way without using the non-resonant process Compton effect in shielding-, absorbermaterial and in target on Resonant flux 6 -10 -2009, Hanoi, Vietnam
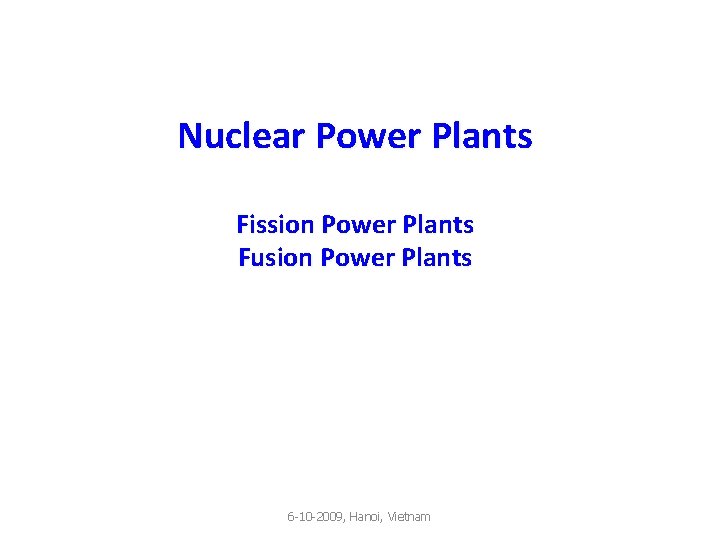
Nuclear Power Plants Fission Power Plants Fusion Power Plants 6 -10 -2009, Hanoi, Vietnam
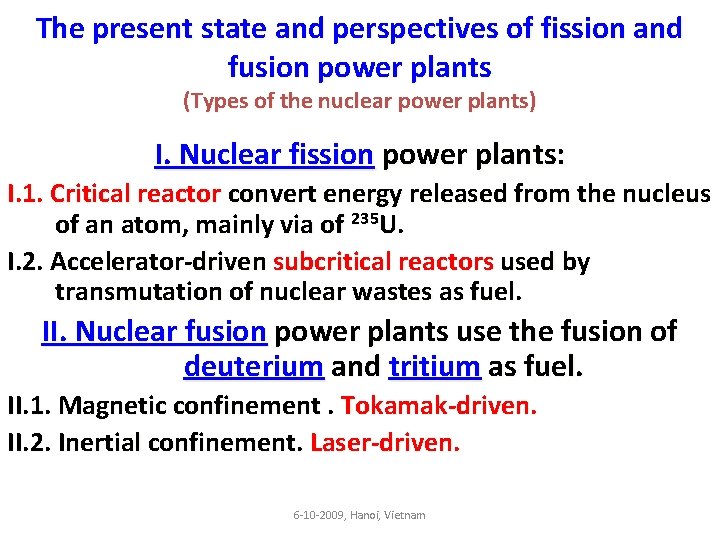
The present state and perspectives of fission and fusion power plants (Types of the nuclear power plants) I. Nuclear fission power plants: I. 1. Critical reactor convert energy released from the nucleus of an atom, mainly via of 235 U. I. 2. Accelerator-driven subcritical reactors used by transmutation of nuclear wastes as fuel. II. Nuclear fusion power plants use the fusion of deuterium and tritium as fuel. II. 1. Magnetic confinement. Tokamak-driven. II. 2. Inertial confinement. Laser-driven. 6 -10 -2009, Hanoi, Vietnam
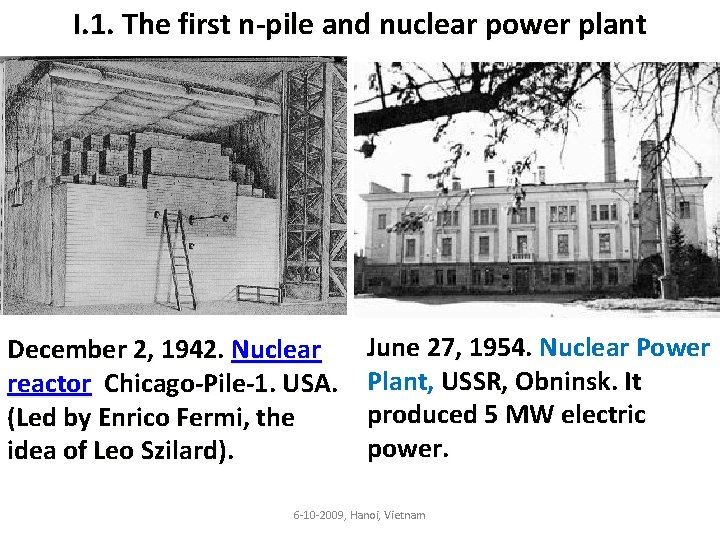
I. 1. The first n-pile and nuclear power plant December 2, 1942. Nuclear reactor Chicago-Pile-1. USA. (Led by Enrico Fermi, the idea of Leo Szilard). June 27, 1954. Nuclear Power Plant, USSR, Obninsk. It produced 5 MW electric power. 6 -10 -2009, Hanoi, Vietnam
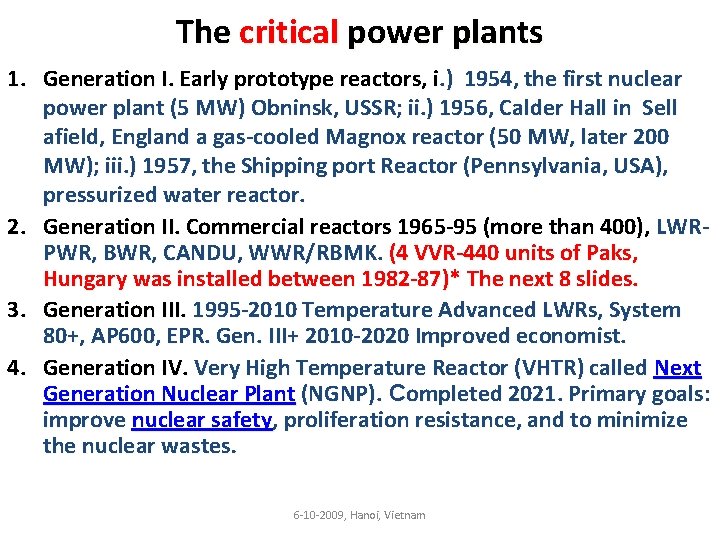
The critical power plants 1. Generation I. Early prototype reactors, i. ) 1954, the first nuclear power plant (5 MW) Obninsk, USSR; ii. ) 1956, Calder Hall in Sell afield, England a gas-cooled Magnox reactor (50 MW, later 200 MW); iii. ) 1957, the Shipping port Reactor (Pennsylvania, USA), pressurized water reactor. 2. Generation II. Commercial reactors 1965 -95 (more than 400), LWRPWR, BWR, CANDU, WWR/RBMK. (4 VVR-440 units of Paks, Hungary was installed between 1982 -87)* The next 8 slides. 3. Generation III. 1995 -2010 Temperature Advanced LWRs, System 80+, AP 600, EPR. Gen. III+ 2010 -2020 Improved economist. 4. Generation IV. Very High Temperature Reactor (VHTR) called Next Generation Nuclear Plant (NGNP). Completed 2021. Primary goals: improve nuclear safety, proliferation resistance, and to minimize the nuclear wastes. 6 -10 -2009, Hanoi, Vietnam
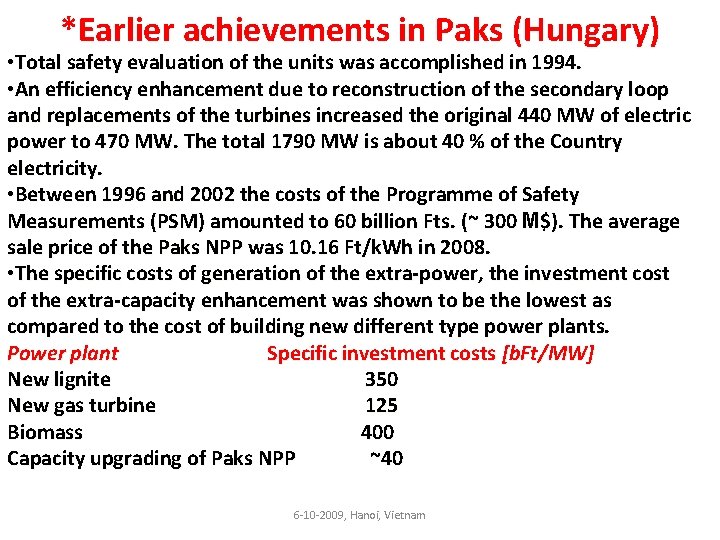
*Earlier achievements in Paks (Hungary) • Total safety evaluation of the units was accomplished in 1994. • An efficiency enhancement due to reconstruction of the secondary loop and replacements of the turbines increased the original 440 MW of electric power to 470 MW. The total 1790 MW is about 40 % of the Country electricity. • Between 1996 and 2002 the costs of the Programme of Safety Measurements (PSM) amounted to 60 billion Fts. (~ 300 M$). The average sale price of the Paks NPP was 10. 16 Ft/k. Wh in 2008. • The specific costs of generation of the extra-power, the investment cost of the extra-capacity enhancement was shown to be the lowest as compared to the cost of building new different type power plants. Power plant Specific investment costs [b. Ft/MW] New lignite 350 New gas turbine 125 Biomass 400 Capacity upgrading of Paks NPP ~40 6 -10 -2009, Hanoi, Vietnam
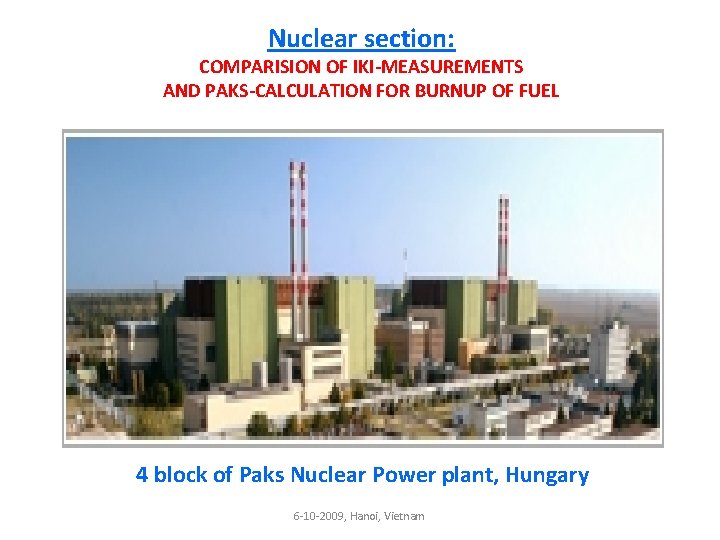
Nuclear section: COMPARISION OF IKI-MEASUREMENTS AND PAKS-CALCULATION FOR BURNUP OF FUEL 4 block of Paks Nuclear Power plant, Hungary 6 -10 -2009, Hanoi, Vietnam
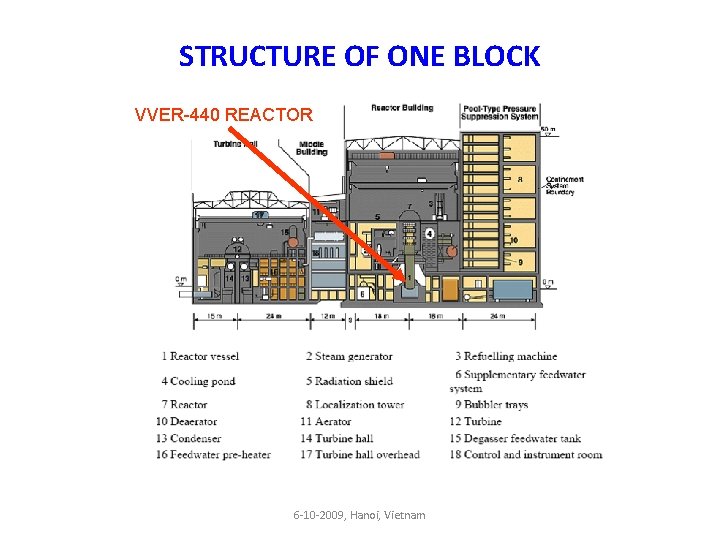
STRUCTURE OF ONE BLOCK VVER-440 REACTOR 6 -10 -2009, Hanoi, Vietnam
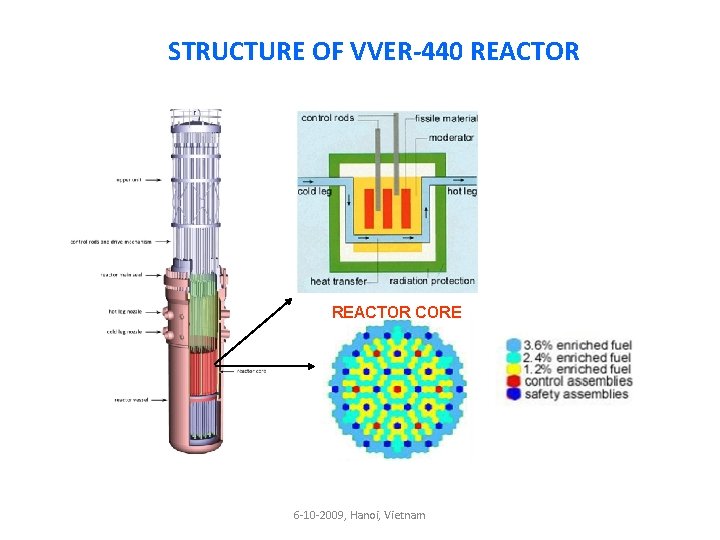
STRUCTURE OF VVER-440 REACTOR CORE 6 -10 -2009, Hanoi, Vietnam
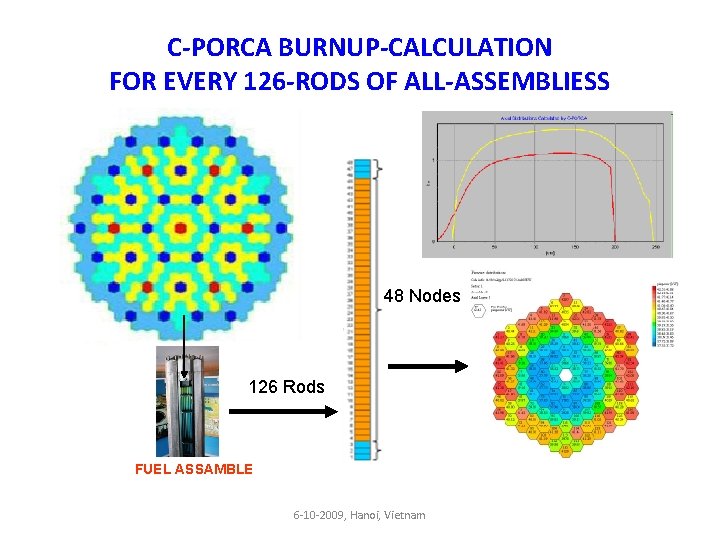
C-PORCA BURNUP-CALCULATION FOR EVERY 126 -RODS OF ALL-ASSEMBLIESS 48 Nodes 126 Rods FUEL ASSAMBLE 6 -10 -2009, Hanoi, Vietnam
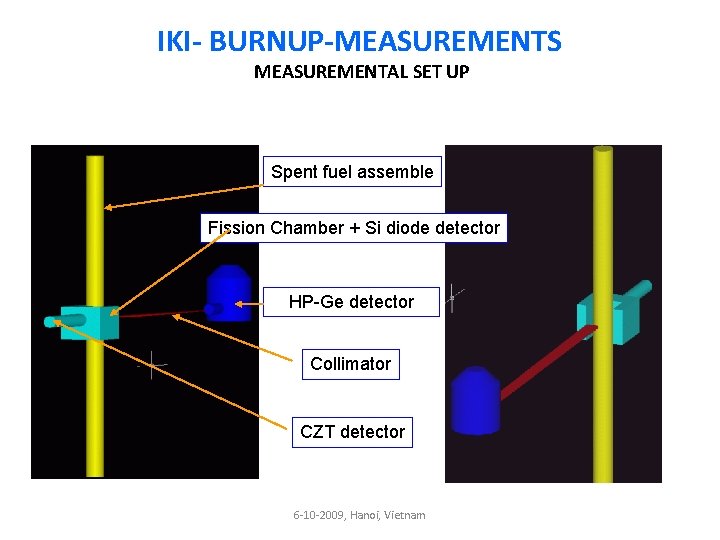
IKI- BURNUP-MEASUREMENTS MEASUREMENTAL SET UP Spent fuel assemble Fission Chamber + Si diode detector HP-Ge detector Collimator CZT detector 6 -10 -2009, Hanoi, Vietnam
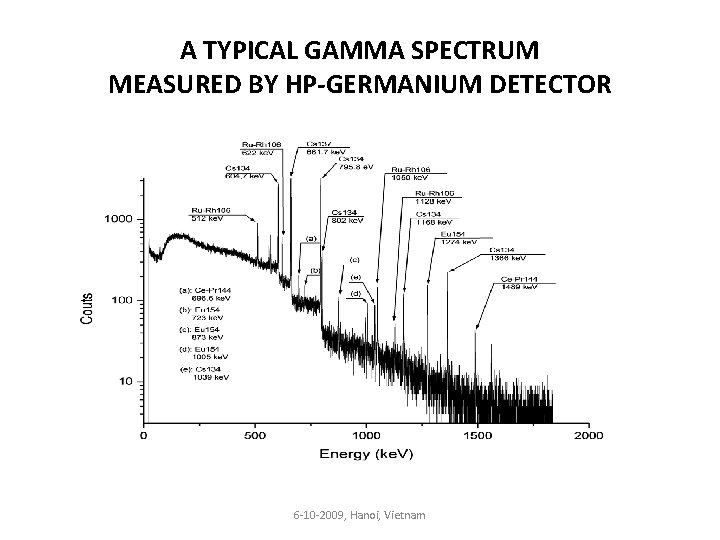
A TYPICAL GAMMA SPECTRUM MEASURED BY HP-GERMANIUM DETECTOR 6 -10 -2009, Hanoi, Vietnam
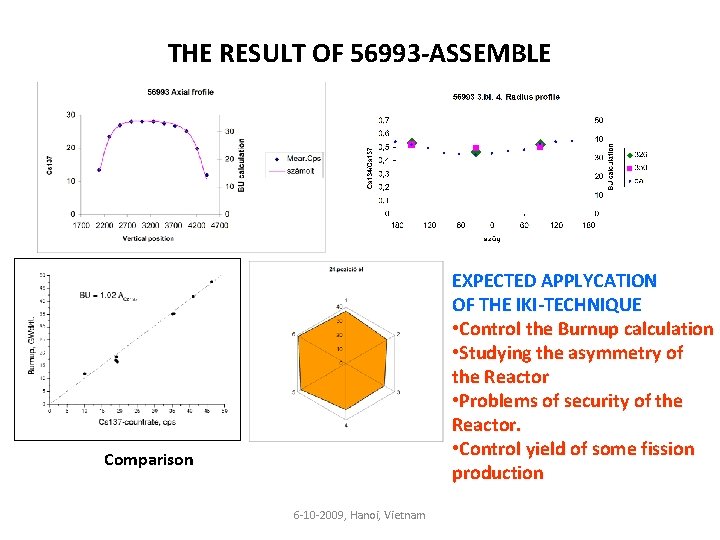
THE RESULT OF 56993 -ASSEMBLE EXPECTED APPLYCATION OF THE IKI-TECHNIQUE • Control the Burnup calculation • Studying the asymmetry of the Reactor • Problems of security of the Reactor. • Control yield of some fission production Comparison 6 -10 -2009, Hanoi, Vietnam
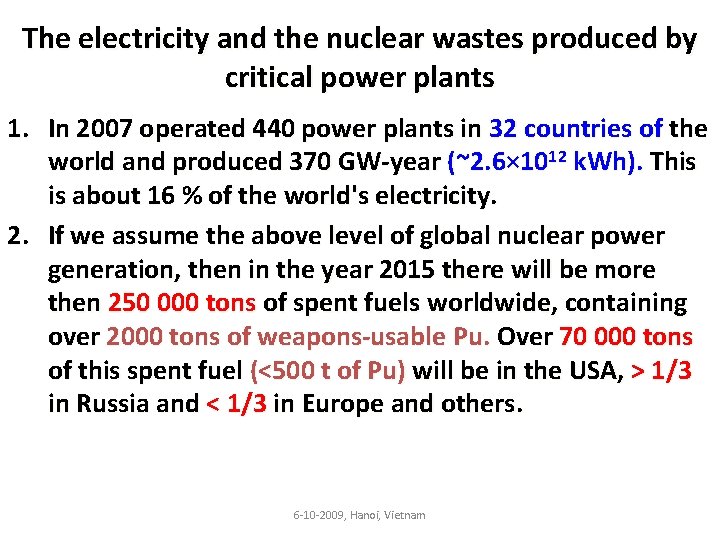
The electricity and the nuclear wastes produced by critical power plants 1. In 2007 operated 440 power plants in 32 countries of the world and produced 370 GW-year (~2. 6× 1012 k. Wh). This is about 16 % of the world's electricity. 2. If we assume the above level of global nuclear power generation, then in the year 2015 there will be more then 250 000 tons of spent fuels worldwide, containing over 2000 tons of weapons-usable Pu. Over 70 000 tons of this spent fuel (<500 t of Pu) will be in the USA, > 1/3 in Russia and < 1/3 in Europe and others. 6 -10 -2009, Hanoi, Vietnam
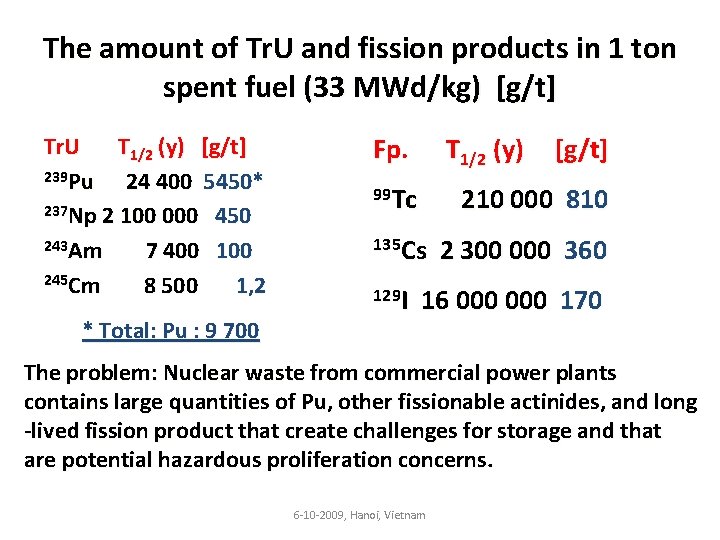
The amount of Tr. U and fission products in 1 ton spent fuel (33 MWd/kg) [g/t] Tr. U T 1/2 (y) [g/t] 239 Pu 24 400 5450* 237 Np 2 100 000 450 243 Am 7 400 100 245 Cm 8 500 1, 2 * Total: Pu : 9 700 Fp. T 1/2 (y) [g/t] 99 Tc 210 000 810 135 Cs 2 300 000 360 129 I 16 000 170 The problem: Nuclear waste from commercial power plants contains large quantities of Pu, other fissionable actinides, and long -lived fission product that create challenges for storage and that are potential hazardous proliferation concerns. 6 -10 -2009, Hanoi, Vietnam
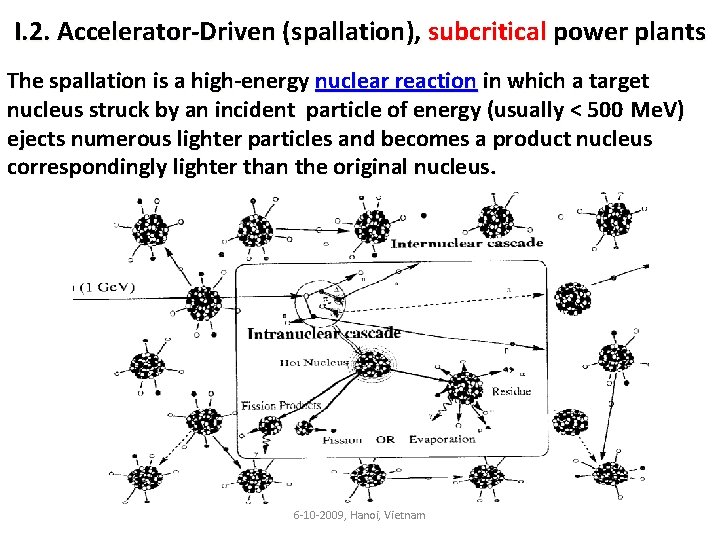
I. 2. Accelerator-Driven (spallation), subcritical power plants The spallation is a high-energy nuclear reaction in which a target nucleus struck by an incident particle of energy (usually < 500 Me. V) ejects numerous lighter particles and becomes a product nucleus correspondingly lighter than the original nucleus. 6 -10 -2009, Hanoi, Vietnam
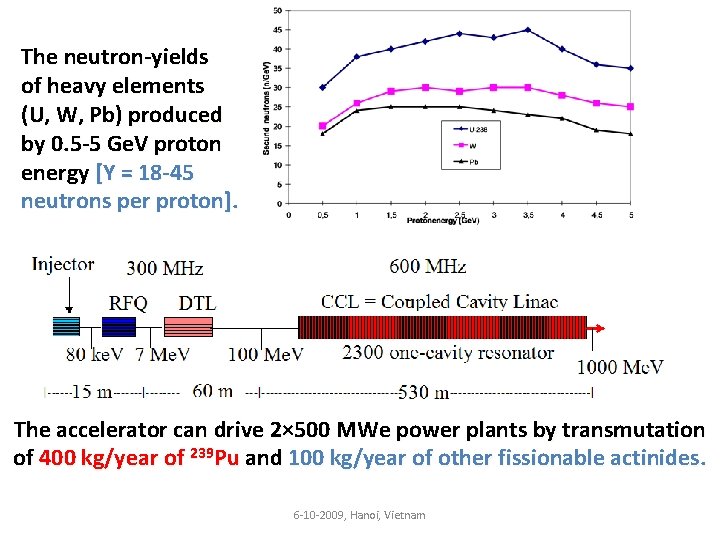
The neutron-yields of heavy elements (U, W, Pb) produced by 0. 5 -5 Ge. V proton energy [Y = 18 -45 neutrons per proton]. The accelerator can drive 2× 500 MWe power plants by transmutation of 400 kg/year of 239 Pu and 100 kg/year of other fissionable actinides. 6 -10 -2009, Hanoi, Vietnam
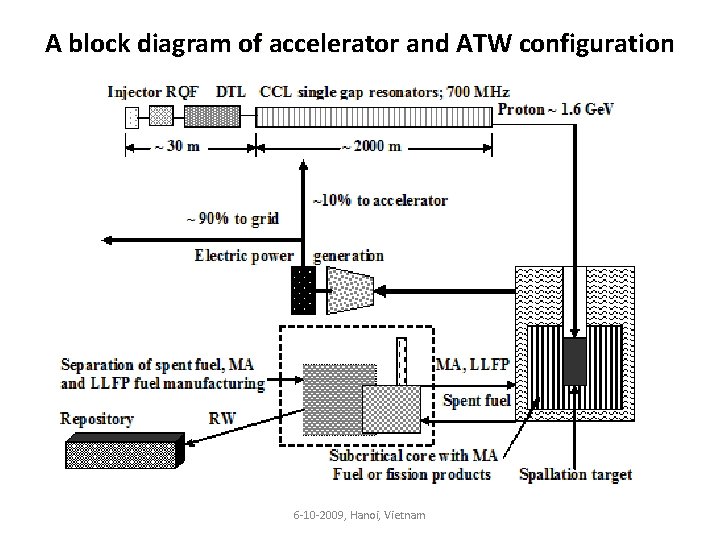
A block diagram of accelerator and ATW configuration 6 -10 -2009, Hanoi, Vietnam
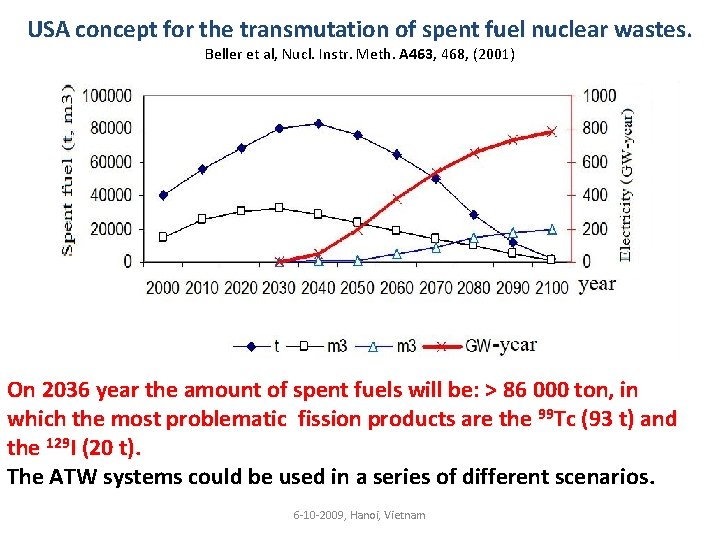
USA concept for the transmutation of spent fuel nuclear wastes. Beller et al, Nucl. Instr. Meth. A 463, 468, (2001) On 2036 year the amount of spent fuels will be: > 86 000 ton, in which the most problematic fission products are the 99 Tc (93 t) and the 129 I (20 t). The ATW systems could be used in a series of different scenarios. 6 -10 -2009, Hanoi, Vietnam
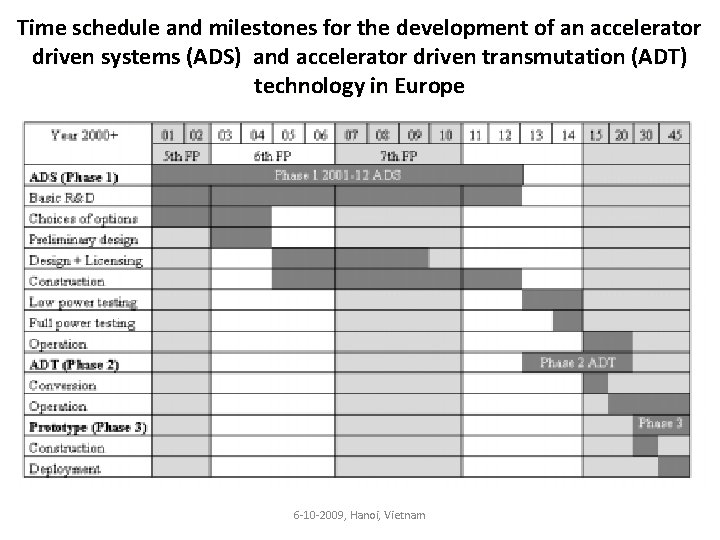
Time schedule and milestones for the development of an accelerator driven systems (ADS) and accelerator driven transmutation (ADT) technology in Europe 6 -10 -2009, Hanoi, Vietnam
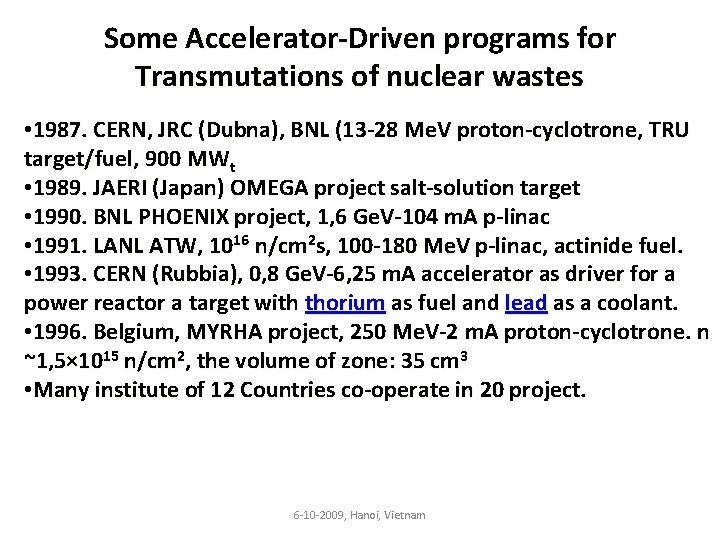
Some Accelerator-Driven programs for Transmutations of nuclear wastes • 1987. CERN, JRC (Dubna), BNL (13 -28 Me. V proton-cyclotrone, TRU target/fuel, 900 MWt • 1989. JAERI (Japan) OMEGA project salt-solution target • 1990. BNL PHOENIX project, 1, 6 Ge. V-104 m. A p-linac • 1991. LANL ATW, 1016 n/cm 2 s, 100 -180 Me. V p-linac, actinide fuel. • 1993. CERN (Rubbia), 0, 8 Ge. V-6, 25 m. A accelerator as driver for a power reactor a target with thorium as fuel and lead as a coolant. • 1996. Belgium, MYRHA project, 250 Me. V-2 m. A proton-cyclotrone. n ~1, 5× 1015 n/cm 2, the volume of zone: 35 cm 3 • Many institute of 12 Countries co-operate in 20 project. 6 -10 -2009, Hanoi, Vietnam
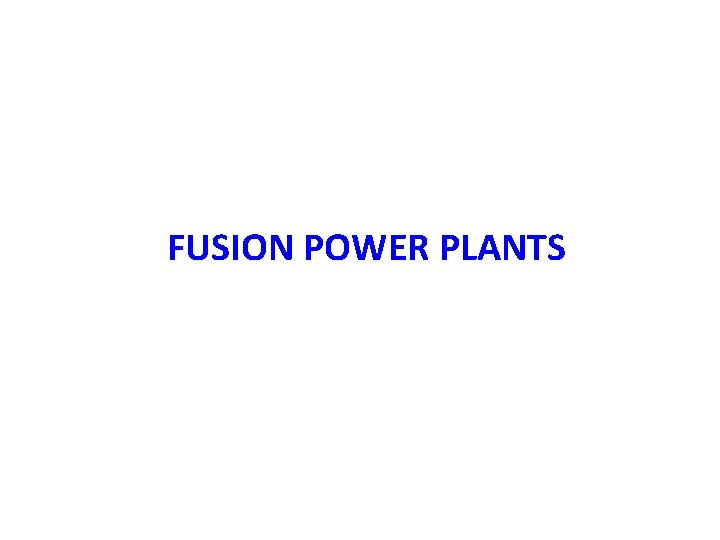
FUSION POWER PLANTS
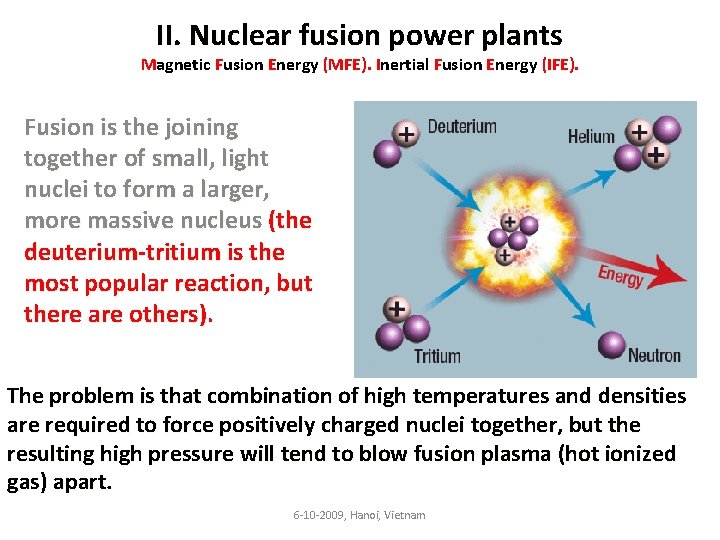
II. Nuclear fusion power plants Magnetic Fusion Energy (MFE). Inertial Fusion Energy (IFE). Fusion is the joining together of small, light nuclei to form a larger, more massive nucleus (the deuterium-tritium is the most popular reaction, but there are others). The problem is that combination of high temperatures and densities are required to force positively charged nuclei together, but the resulting high pressure will tend to blow fusion plasma (hot ionized gas) apart. 6 -10 -2009, Hanoi, Vietnam
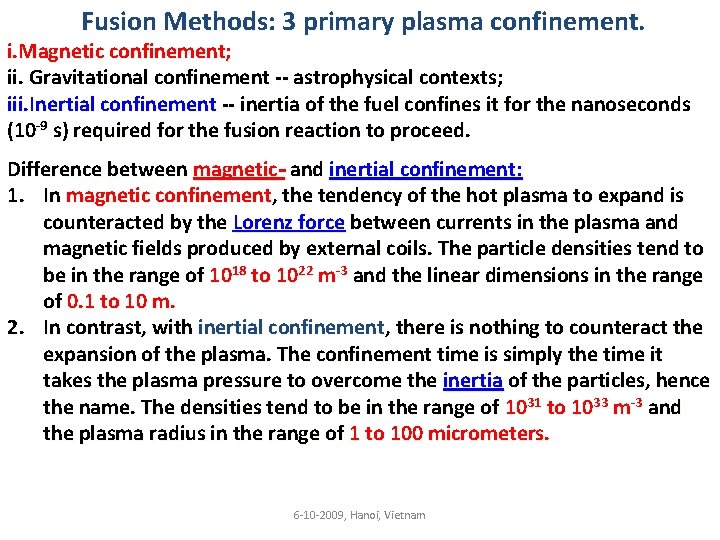
Fusion Methods: 3 primary plasma confinement. i. Magnetic confinement; ii. Gravitational confinement -- astrophysical contexts; iii. Inertial confinement -- inertia of the fuel confines it for the nanoseconds (10 -9 s) required for the fusion reaction to proceed. Difference between magnetic- and inertial confinement: 1. In magnetic confinement, the tendency of the hot plasma to expand is counteracted by the Lorenz force between currents in the plasma and magnetic fields produced by external coils. The particle densities tend to be in the range of 1018 to 1022 m-3 and the linear dimensions in the range of 0. 1 to 10 m. 2. In contrast, with inertial confinement, there is nothing to counteract the expansion of the plasma. The confinement time is simply the time it takes the plasma pressure to overcome the inertia of the particles, hence the name. The densities tend to be in the range of 1031 to 1033 m-3 and the plasma radius in the range of 1 to 100 micrometers. 6 -10 -2009, Hanoi, Vietnam
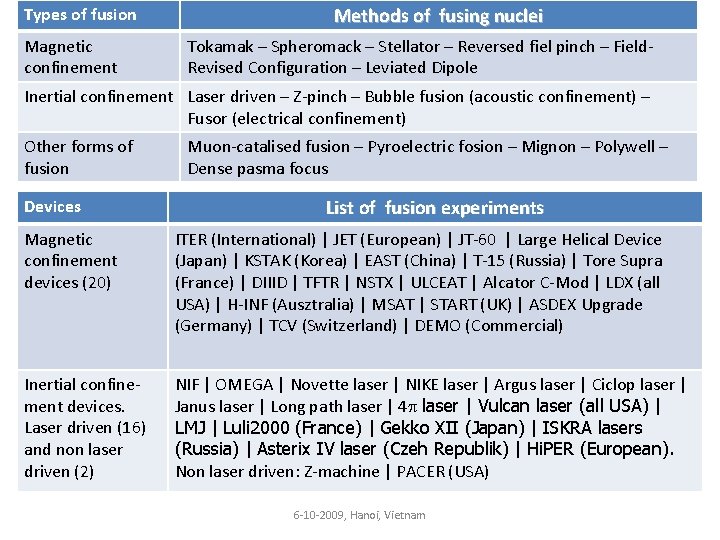
Types of fusion Magnetic confinement Methods of fusing nuclei Tokamak – Spheromack – Stellator – Reversed fiel pinch – Field. Revised Configuration – Leviated Dipole Inertial confinement Laser driven – Z-pinch – Bubble fusion (acoustic confinement) – Fusor (electrical confinement) Other forms of fusion Devices Muon-catalised fusion – Pyroelectric fosion – Mignon – Polywell – Dense pasma focus List of fusion experiments Magnetic confinement devices (20) ITER (International) | JET (European) | JT-60 | Large Helical Device (Japan) | KSTAK (Korea) | EAST (China) | T-15 (Russia) | Tore Supra (France) | DIIID | TFTR | NSTX | ULCEAT | Alcator C-Mod | LDX (all USA) | H-INF (Ausztralia) | MSAT | START (UK) | ASDEX Upgrade (Germany) | TCV (Switzerland) | DEMO (Commercial) Inertial confinement devices. Laser driven (16) and non laser driven (2) NIF | OMEGA | Novette laser | NIKE laser | Argus laser | Ciclop laser | Janus laser | Long path laser | 4 p laser | Vulcan laser (all USA) | LMJ | Luli 2000 (France) | Gekko XII (Japan) | ISKRA lasers (Russia) | Asterix IV laser (Czeh Republik) | Hi. PER (European). Non laser driven: Z-machine | PACER (USA) 6 -10 -2009, Hanoi, Vietnam
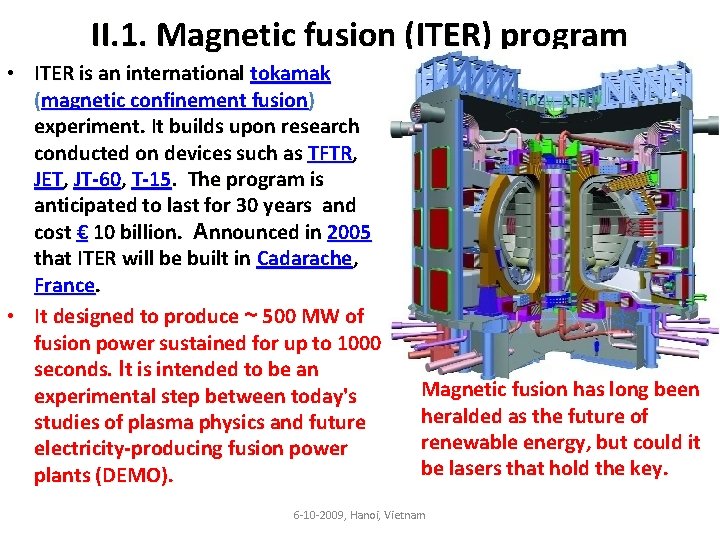
II. 1. Magnetic fusion (ITER) program • ITER is an international tokamak (magnetic confinement fusion) experiment. It builds upon research conducted on devices such as TFTR, JET, JT-60, T-15. The program is anticipated to last for 30 years and cost € 10 billion. Announced in 2005 that ITER will be built in Cadarache, France. • It designed to produce ~ 500 MW of fusion power sustained for up to 1000 seconds. It is intended to be an experimental step between today's studies of plasma physics and future electricity-producing fusion power plants (DEMO). Magnetic fusion has long been heralded as the future of renewable energy, but could it be lasers that hold the key. 6 -10 -2009, Hanoi, Vietnam
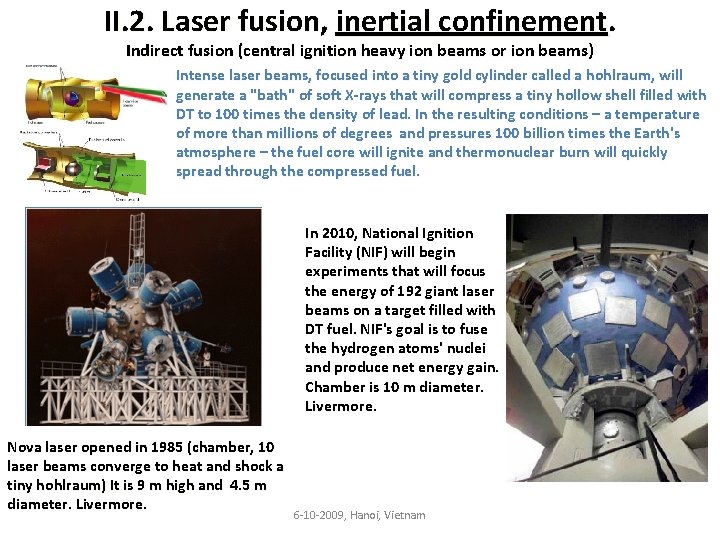
II. 2. Laser fusion, inertial confinement. Indirect fusion (central ignition heavy ion beams or ion beams) Intense laser beams, focused into a tiny gold cylinder called a hohlraum, will generate a "bath" of soft X-rays that will compress a tiny hollow shell filled with DT to 100 times the density of lead. In the resulting conditions – a temperature of more than millions of degrees and pressures 100 billion times the Earth's atmosphere – the fuel core will ignite and thermonuclear burn will quickly spread through the compressed fuel. In 2010, National Ignition Facility (NIF) will begin experiments that will focus the energy of 192 giant laser beams on a target filled with DT fuel. NIF's goal is to fuse the hydrogen atoms' nuclei and produce net energy gain. Chamber is 10 m diameter. Livermore. Nova laser opened in 1985 (chamber, 10 laser beams converge to heat and shock a tiny hohlraum) It is 9 m high and 4. 5 m diameter. Livermore. 6 -10 -2009, Hanoi, Vietnam
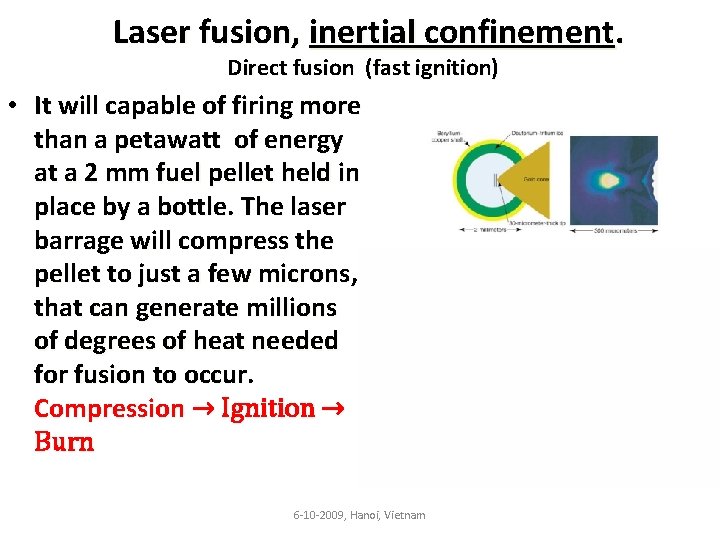
Laser fusion, inertial confinement. Direct fusion (fast ignition) • It will capable of firing more than a petawatt of energy at a 2 mm fuel pellet held in place by a bottle. The laser barrage will compress the pellet to just a few microns, that can generate millions of degrees of heat needed for fusion to occur. Compression → Ignition → Burn 6 -10 -2009, Hanoi, Vietnam
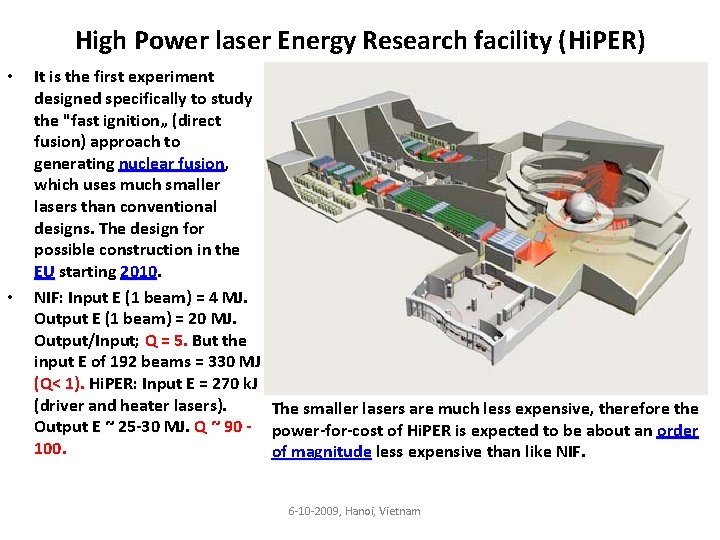
High Power laser Energy Research facility (Hi. PER) • • It is the first experiment designed specifically to study the "fast ignition„ (direct fusion) approach to generating nuclear fusion, which uses much smaller lasers than conventional designs. The design for possible construction in the EU starting 2010. NIF: Input E (1 beam) = 4 MJ. Output E (1 beam) = 20 MJ. Output/Input; Q = 5. But the input E of 192 beams = 330 MJ (Q< 1). Hi. PER: Input E = 270 k. J (driver and heater lasers). Output E ~ 25 -30 MJ. Q ~ 90 100. The smaller lasers are much less expensive, therefore the power-for-cost of Hi. PER is expected to be about an order of magnitude less expensive than like NIF. 6 -10 -2009, Hanoi, Vietnam
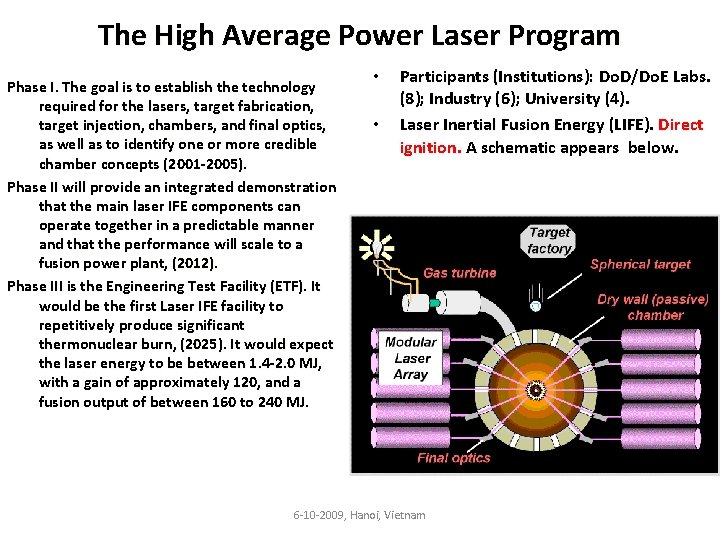
The High Average Power Laser Program Phase I. The goal is to establish the technology required for the lasers, target fabrication, target injection, chambers, and final optics, as well as to identify one or more credible chamber concepts (2001 -2005). Phase II will provide an integrated demonstration that the main laser IFE components can operate together in a predictable manner and that the performance will scale to a fusion power plant, (2012). Phase III is the Engineering Test Facility (ETF). It would be the first Laser IFE facility to repetitively produce significant thermonuclear burn, (2025). It would expect the laser energy to be between 1. 4 -2. 0 MJ, with a gain of approximately 120, and a fusion output of between 160 to 240 MJ. • • Participants (Institutions): Do. D/Do. E Labs. (8); Industry (6); University (4). Laser Inertial Fusion Energy (LIFE). Direct ignition. A schematic appears below. 6 -10 -2009, Hanoi, Vietnam
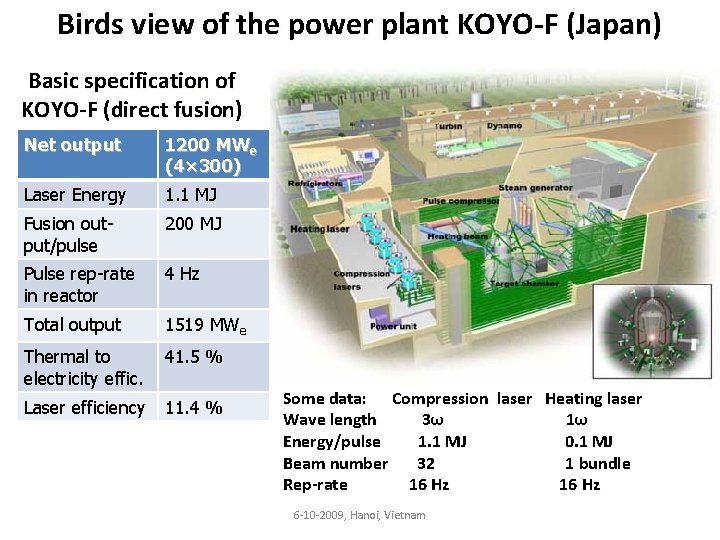
Birds view of the power plant KOYO-F (Japan) Basic specification of KOYO-F (direct fusion) Net output 1200 MWe (4× 300) Laser Energy 1. 1 MJ Fusion output/pulse 200 MJ Pulse rep-rate in reactor 4 Hz Total output 1519 MWe Thermal to electricity effic. 41. 5 % Laser efficiency 11. 4 % Some data: Compression laser Heating laser Wave length 3ω 1ω Energy/pulse 1. 1 MJ 0. 1 MJ Beam number 32 1 bundle Rep-rate 16 Hz 6 -10 -2009, Hanoi, Vietnam
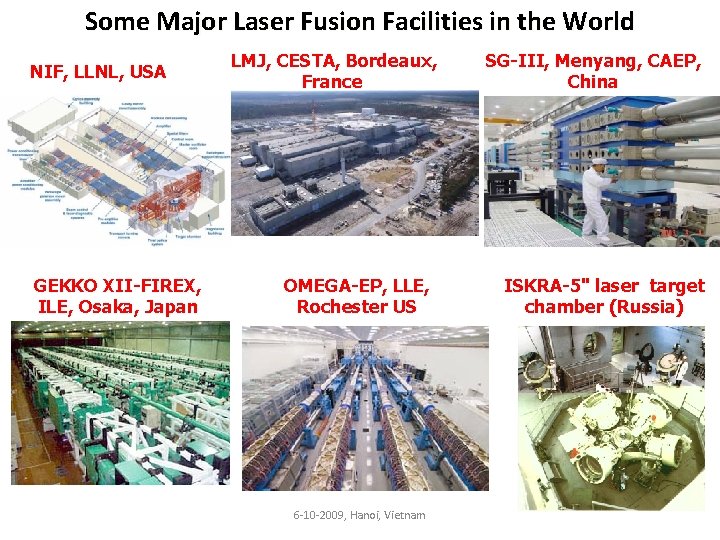
Some Major Laser Fusion Facilities in the World NIF, LLNL, USA GEKKO XII-FIREX, ILE, Osaka, Japan LMJ, CESTA, Bordeaux, France OMEGA-EP, LLE, Rochester US 6 -10 -2009, Hanoi, Vietnam SG-III, Menyang, CAEP, China ISKRA-5" laser target chamber (Russia)
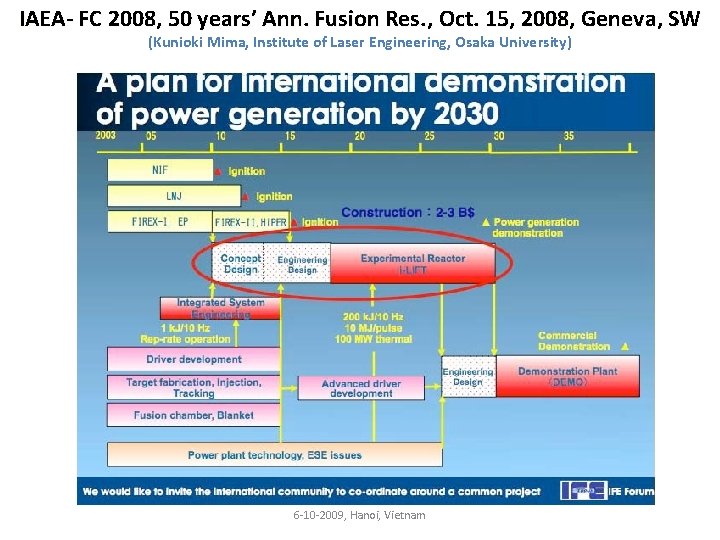
IAEA- FC 2008, 50 years’ Ann. Fusion Res. , Oct. 15, 2008, Geneva, SW (Kunioki Mima, Institute of Laser Engineering, Osaka University) 6 -10 -2009, Hanoi, Vietnam
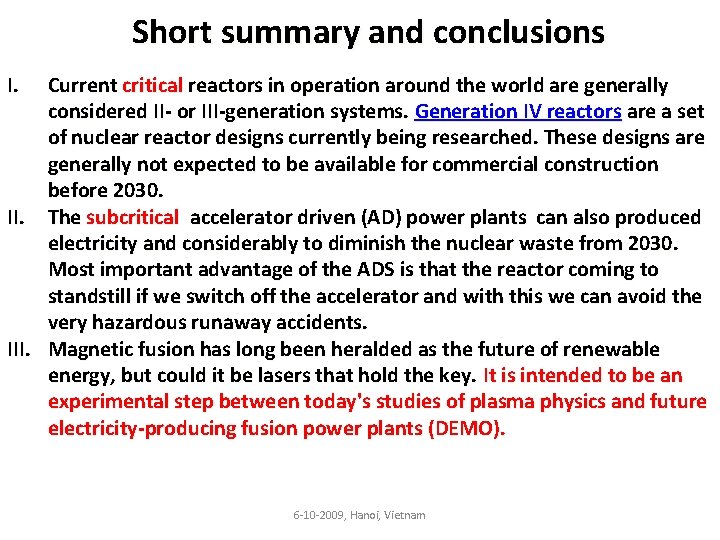
Short summary and conclusions I. Current critical reactors in operation around the world are generally considered II- or III-generation systems. Generation IV reactors are a set of nuclear reactor designs currently being researched. These designs are generally not expected to be available for commercial construction before 2030. II. The subcritical accelerator driven (AD) power plants can also produced electricity and considerably to diminish the nuclear waste from 2030. Most important advantage of the ADS is that the reactor coming to standstill if we switch off the accelerator and with this we can avoid the very hazardous runaway accidents. III. Magnetic fusion has long been heralded as the future of renewable energy, but could it be lasers that hold the key. It is intended to be an experimental step between today's studies of plasma physics and future electricity-producing fusion power plants (DEMO). 6 -10 -2009, Hanoi, Vietnam
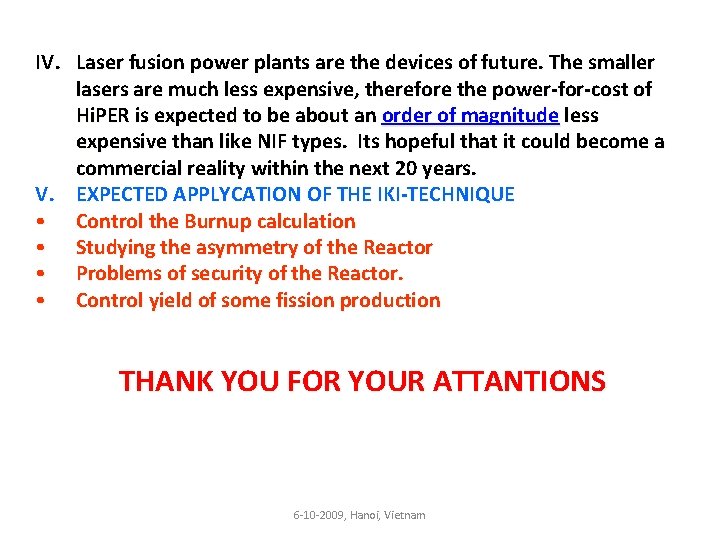
IV. Laser fusion power plants are the devices of future. The smaller lasers are much less expensive, therefore the power-for-cost of Hi. PER is expected to be about an order of magnitude less expensive than like NIF types. Its hopeful that it could become a commercial reality within the next 20 years. V. EXPECTED APPLYCATION OF THE IKI-TECHNIQUE • Control the Burnup calculation • Studying the asymmetry of the Reactor • Problems of security of the Reactor. • Control yield of some fission production THANK YOU FOR YOUR ATTANTIONS 6 -10 -2009, Hanoi, Vietnam
Lesson 15 nuclear quest nuclear reactions
Fisión nuclear vs fision nuclear
Measurement topic
General physics 1 measurements
General physics 1 measurements
Measurements in physics
Physics topic 12
Iupap
Magic nuclei
Skobeltsyn institute of nuclear physics
Nuclear energy
Scattering cross section in nuclear physics
Budker
Petersburg nuclear physics institute
Physics topic 12
Nuclear physics
Nuclear physics topics for presentation
Nuclear physics
Nuclear physics
Nuclear physics
Nuclear physics b
Magic number in nuclear physics
Nuclear physics
Budker
Nuclear physics day
Applied physics yale
Chaos aip
Maritime applied physics corporation
Jhu apl internship
Why does it happen
University physics with modern physics fifteenth edition
Example physics ia
Measurements equivalents and adjustments
Chapter 2 measurements and calculations
Force and torque measurements
Ee8403 measurements and instrumentation
Temperature is an anthropometric measurement
Chapter 37 vital signs and measurements true or false
Angle measure and segment lengths
Body measurements and vital signs chapter 36
Vital signs and anthropometric measurements:
Measurements and their uncertainty
Pharmaceutical measurements
Working principle of tool makers microscope
Metrology and measurements subject code
Measuring abbreviations
How to calculate percent error in chemistry
Pharmacy measurements
Si unit of pressure
Using and expressing measurements
Unit 2 lesson 2 scientific notation
Module 7 weights and measurements
Household conversions chart
Instrumentation and measurements