DARK ENERGY SURVEY DES Francisco Javier Castander Serentill
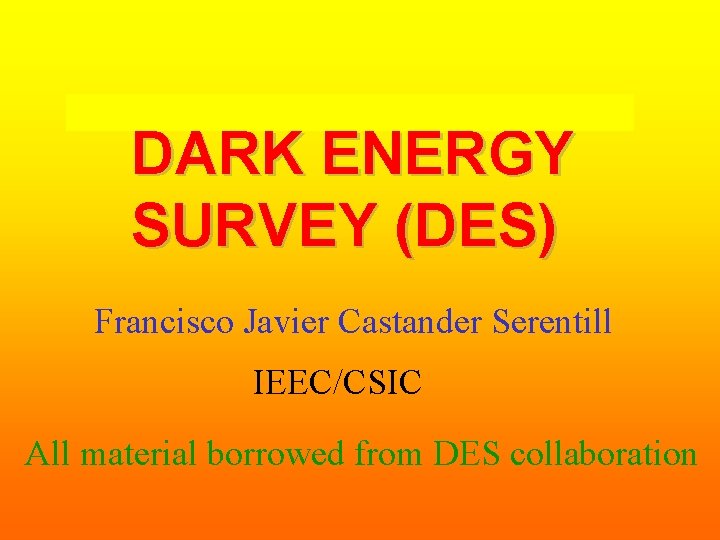
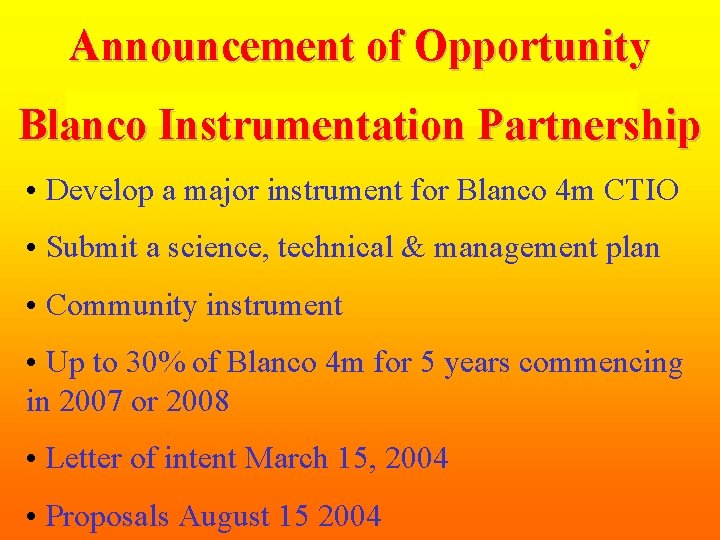
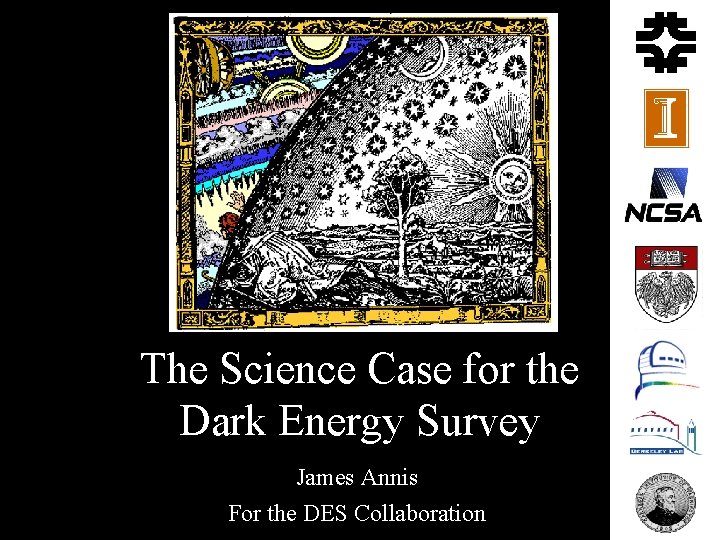
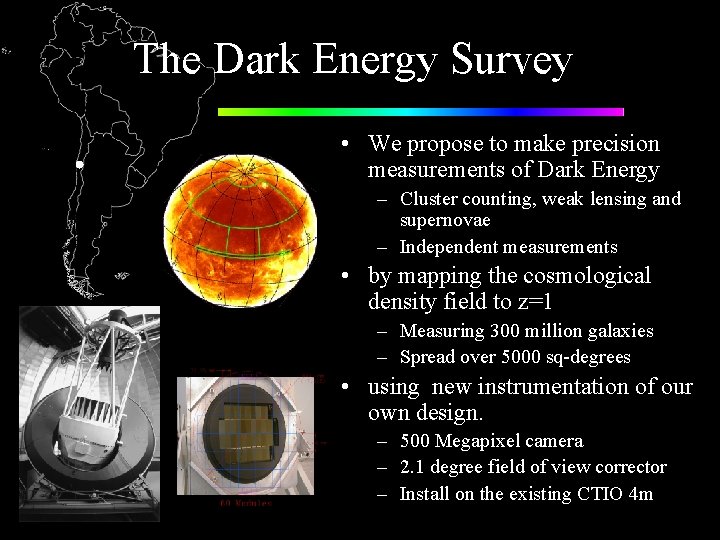
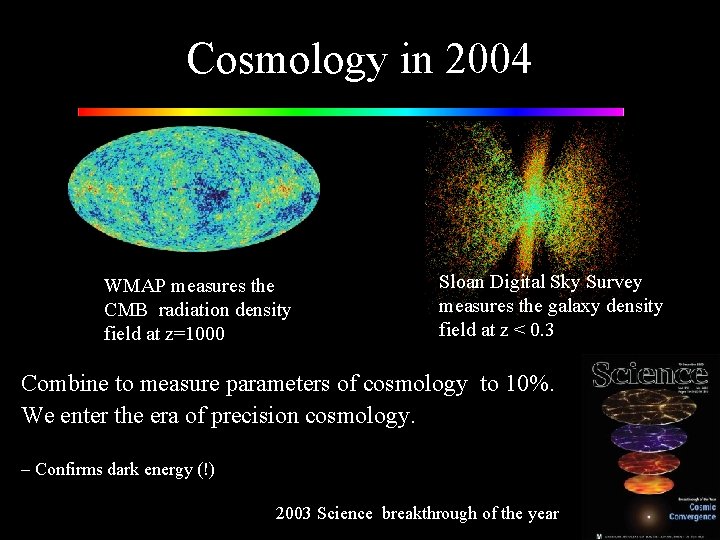
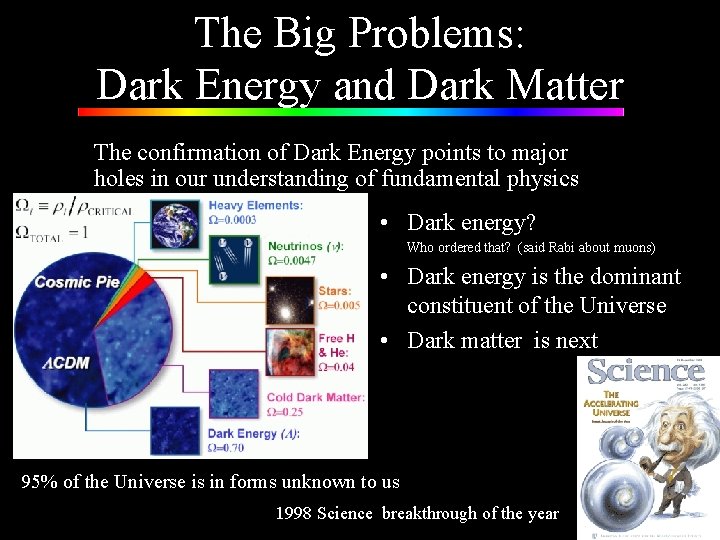
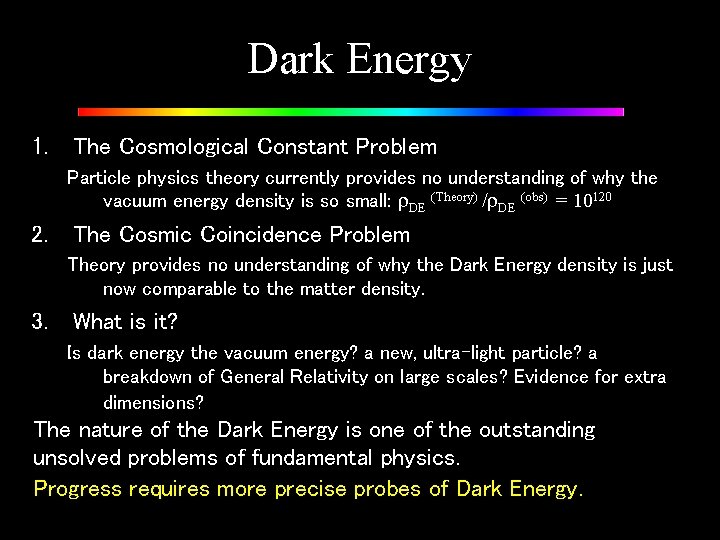
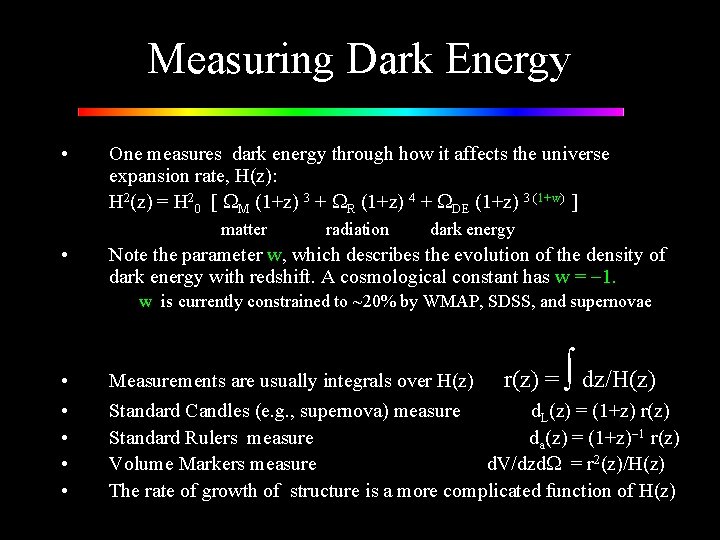
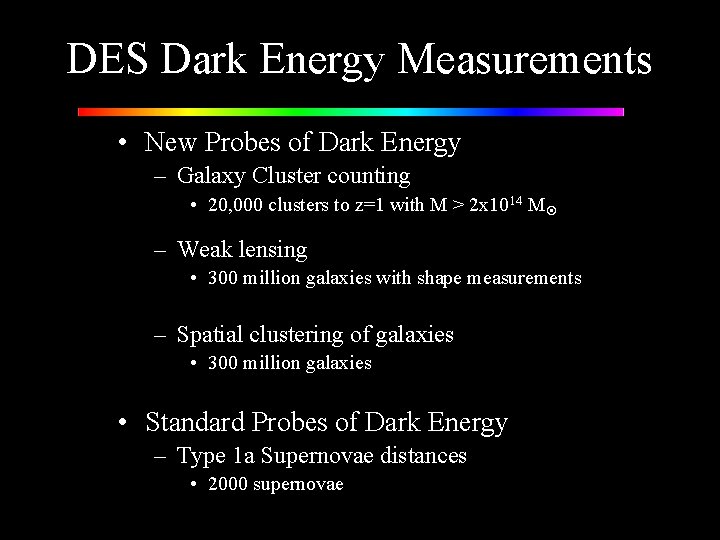
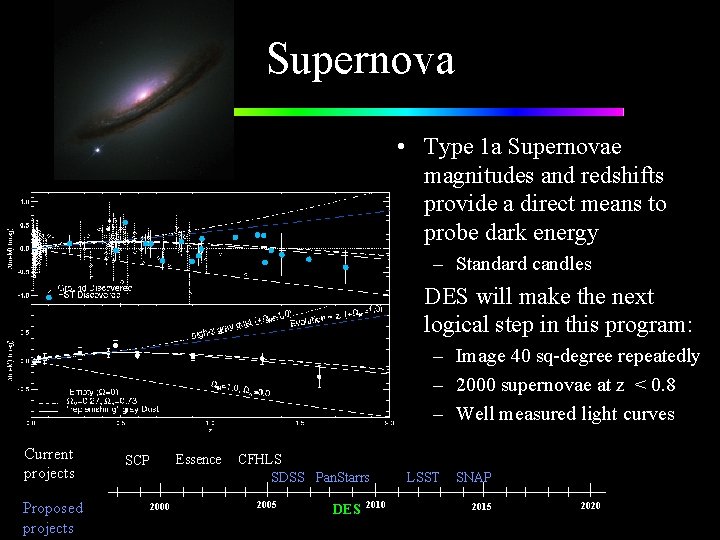
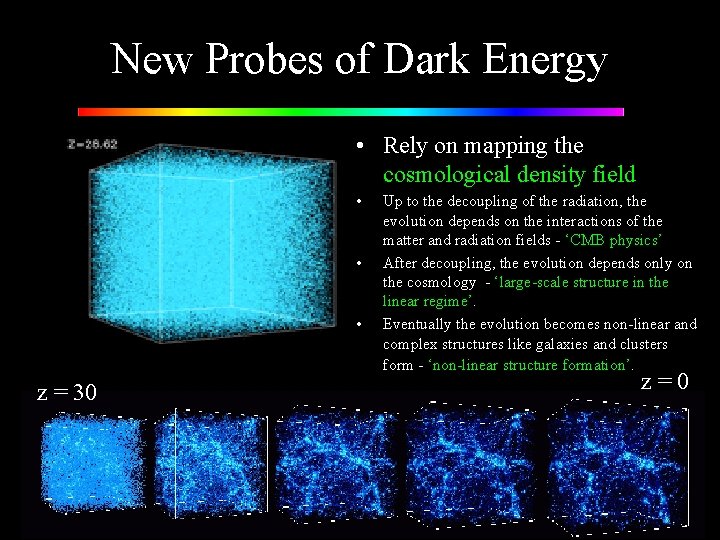
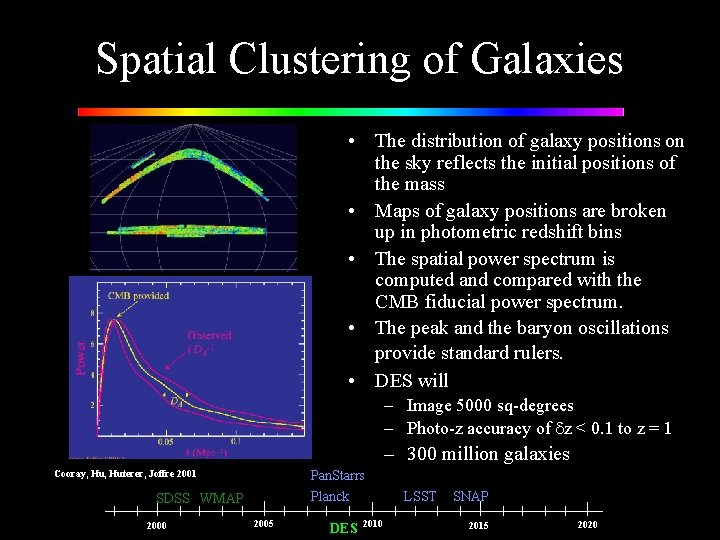
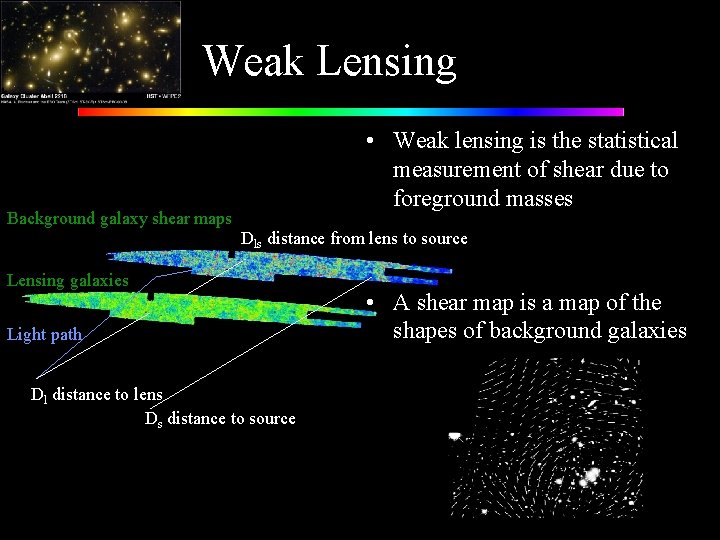
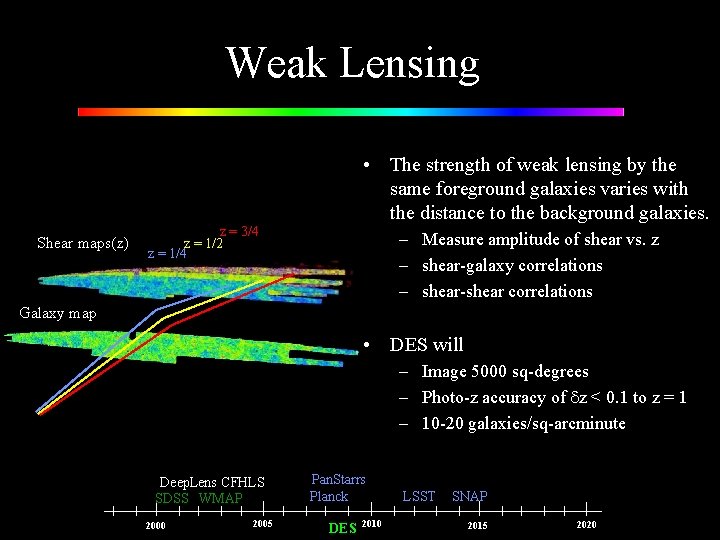
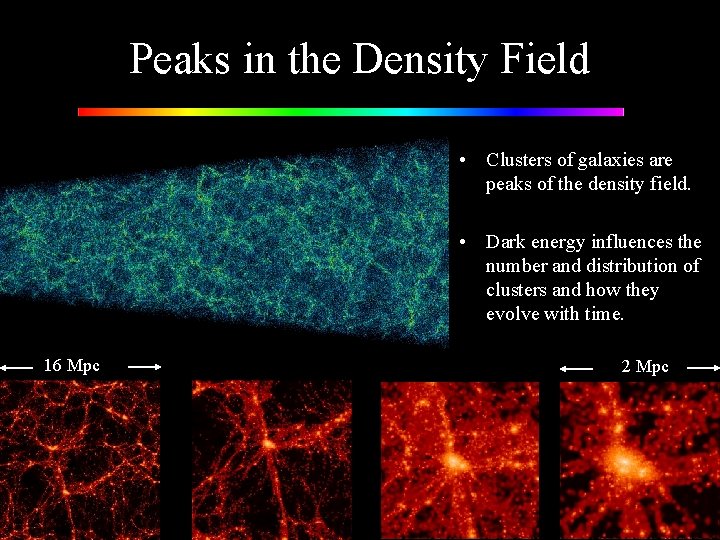
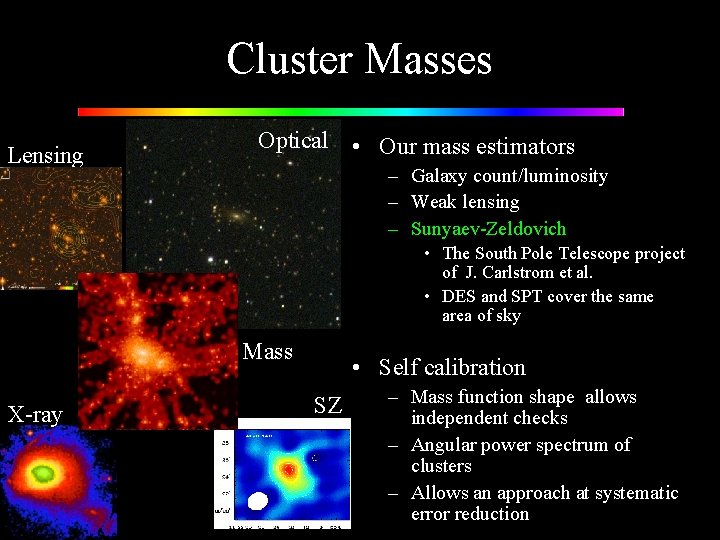
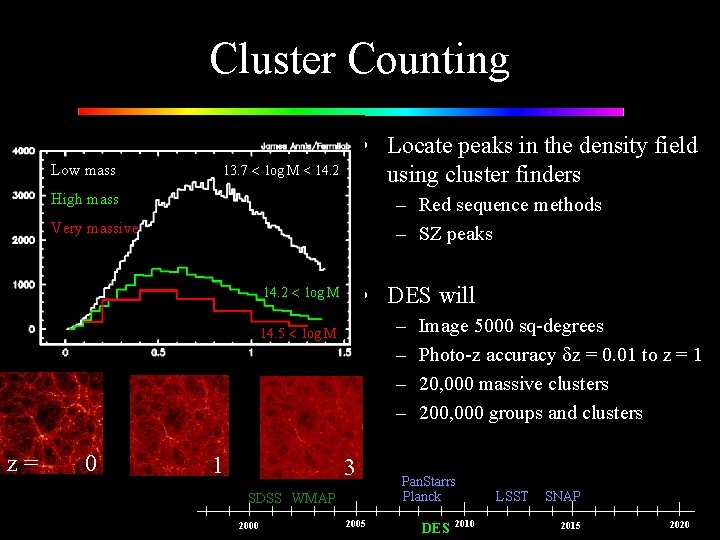
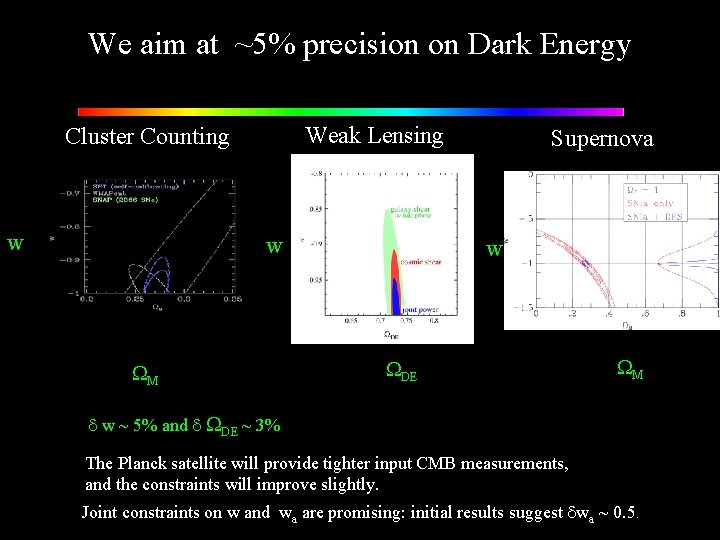
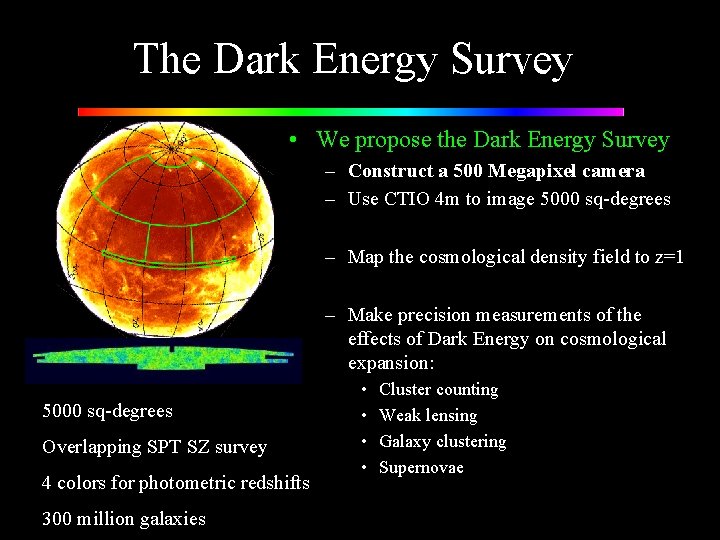
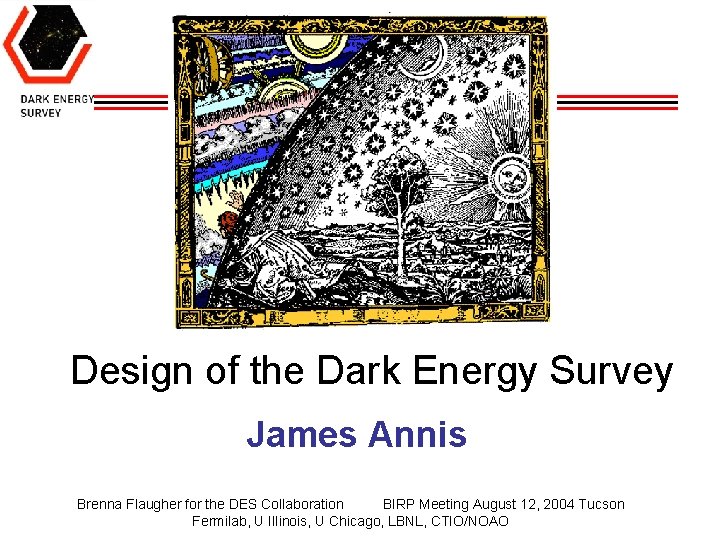
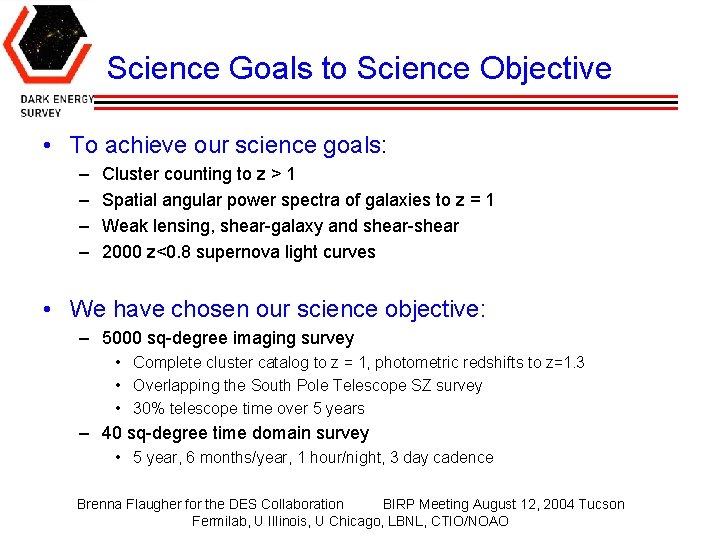
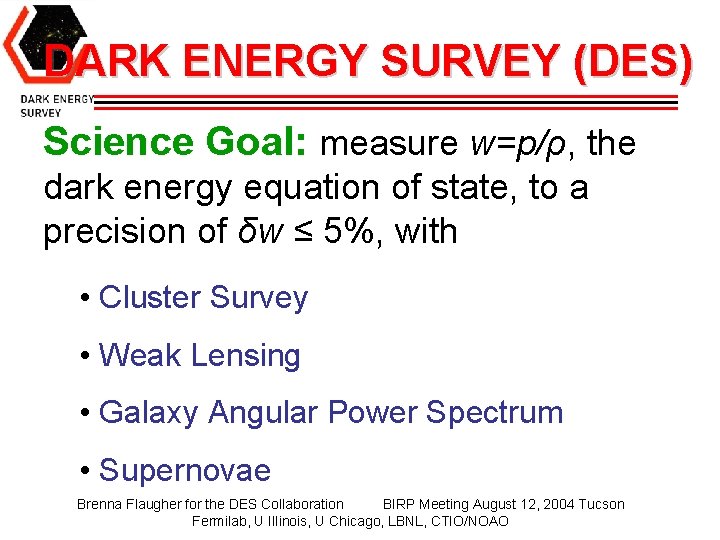
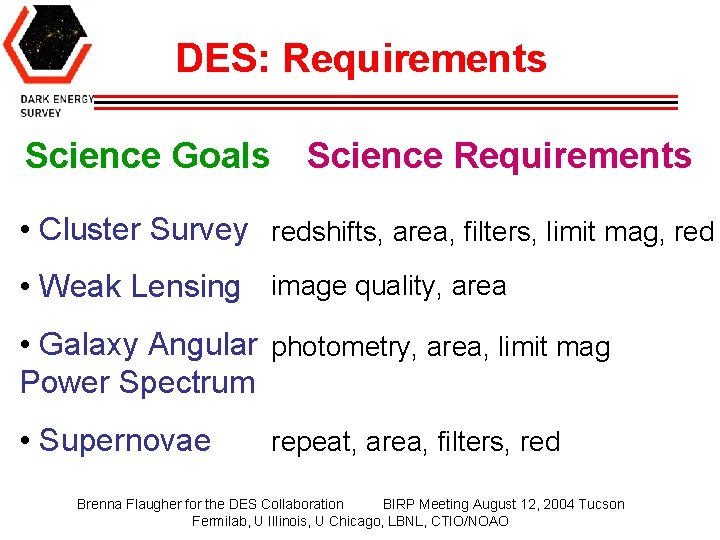
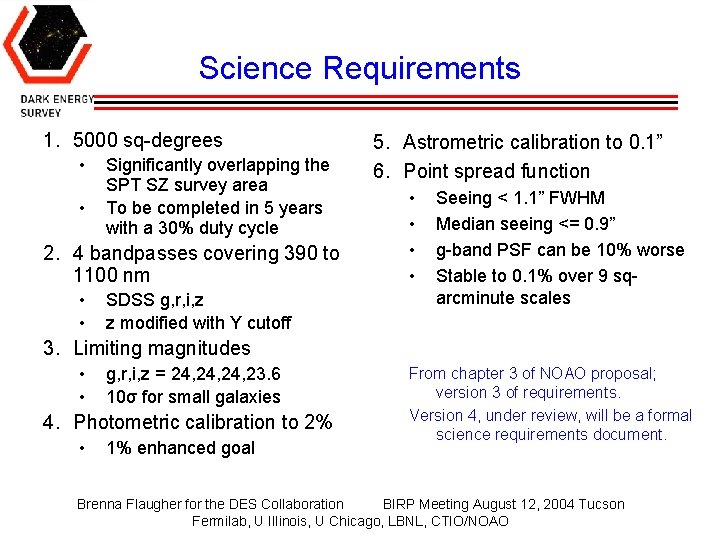
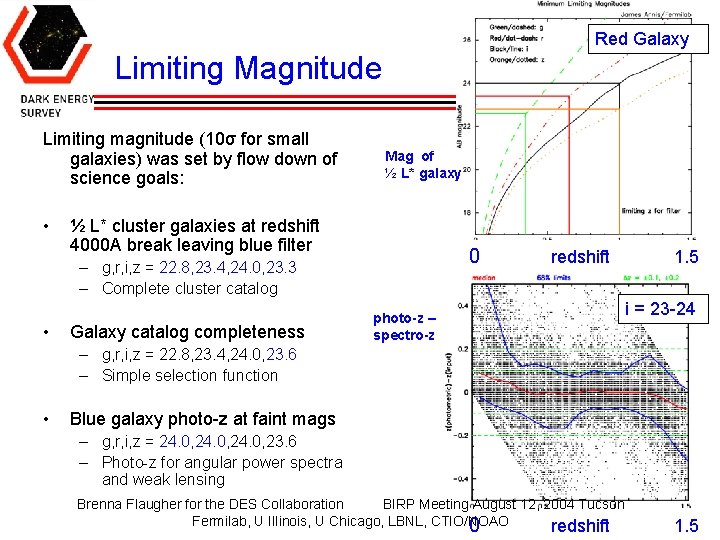
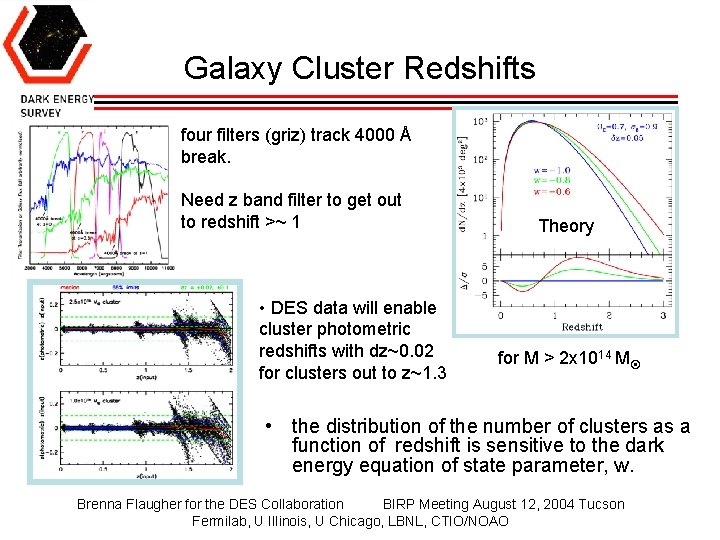
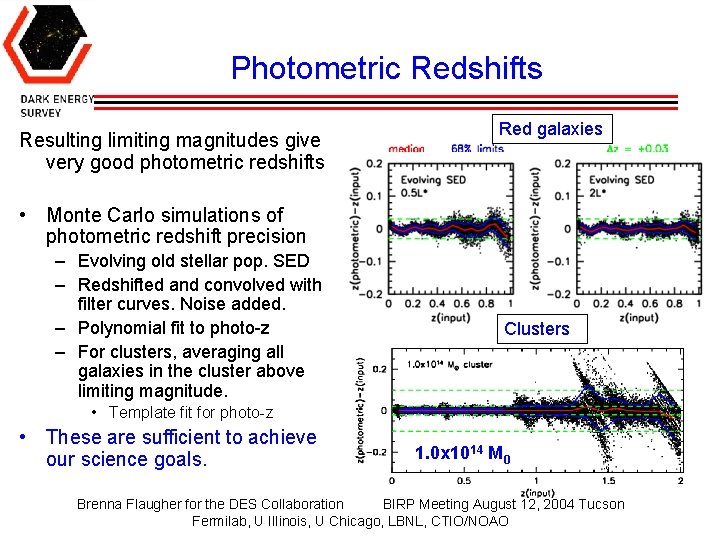
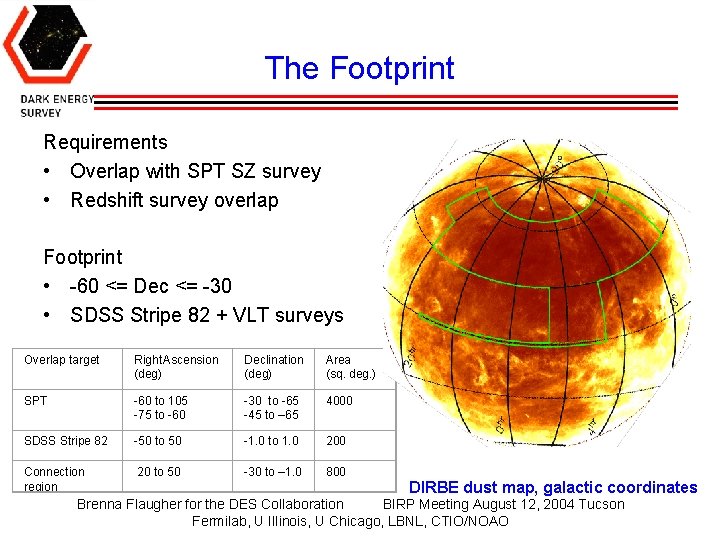
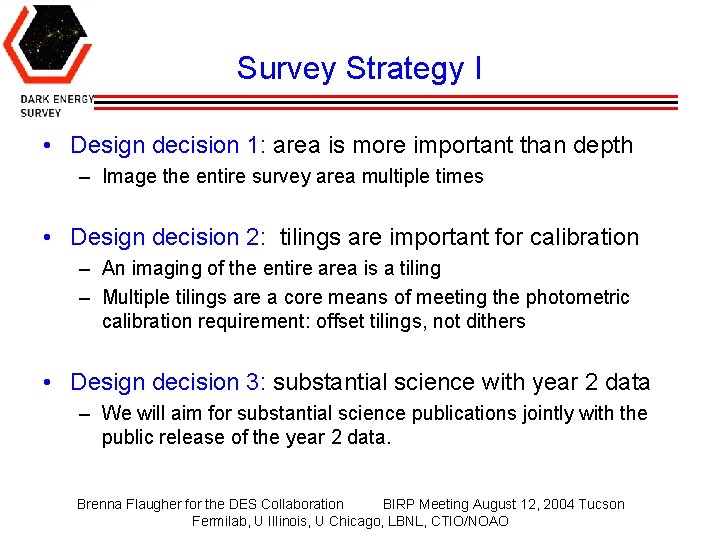
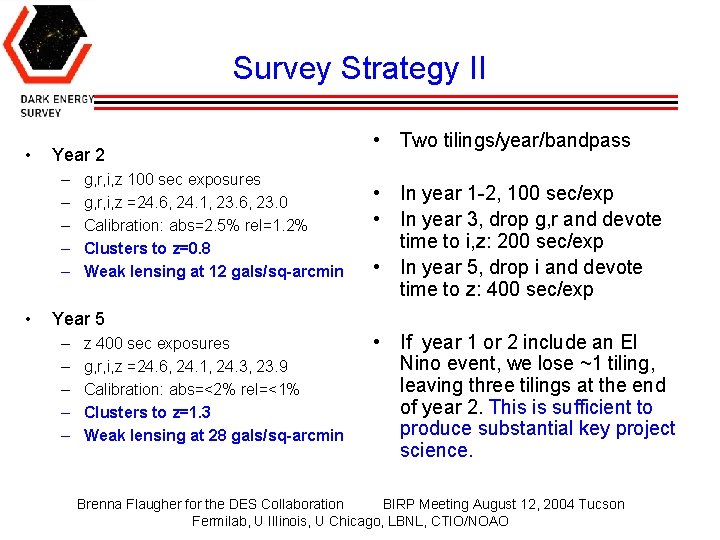
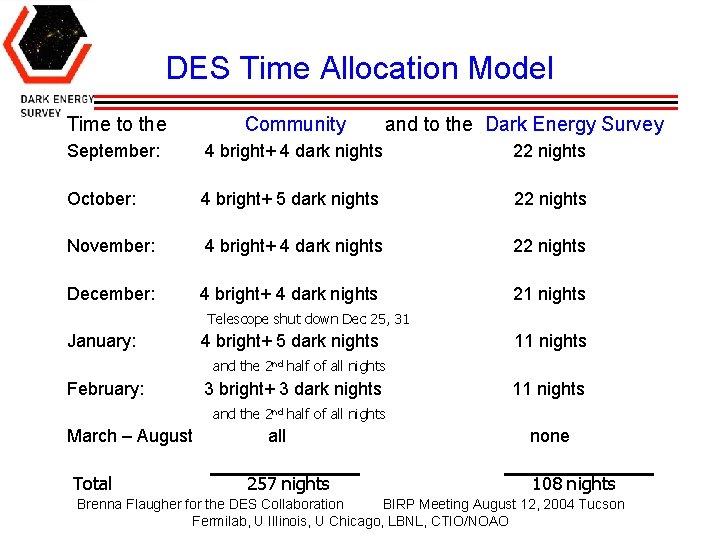
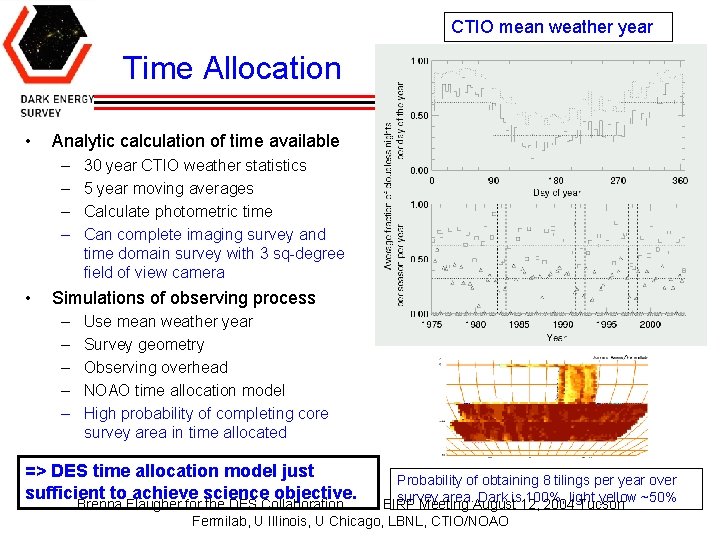
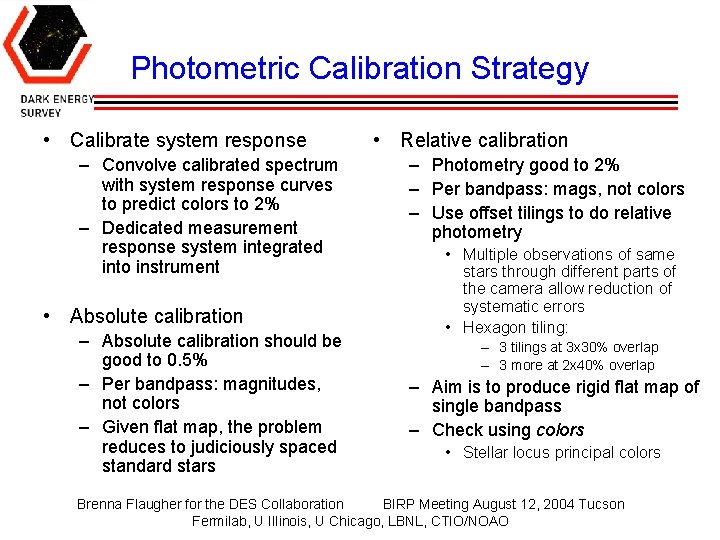
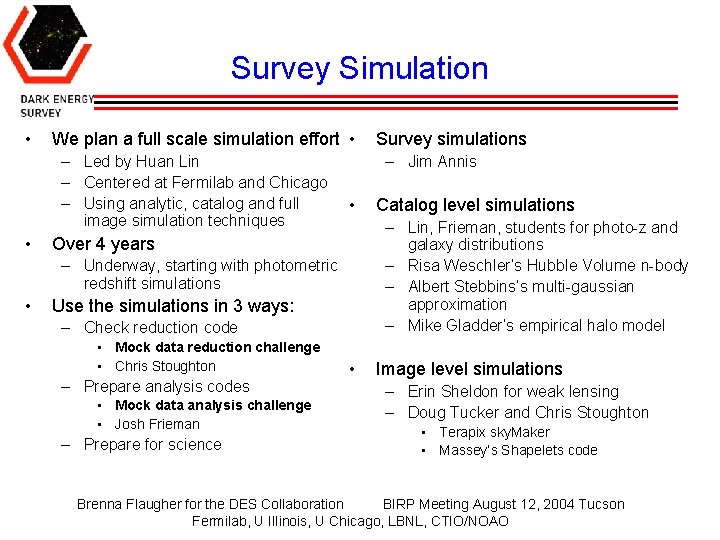
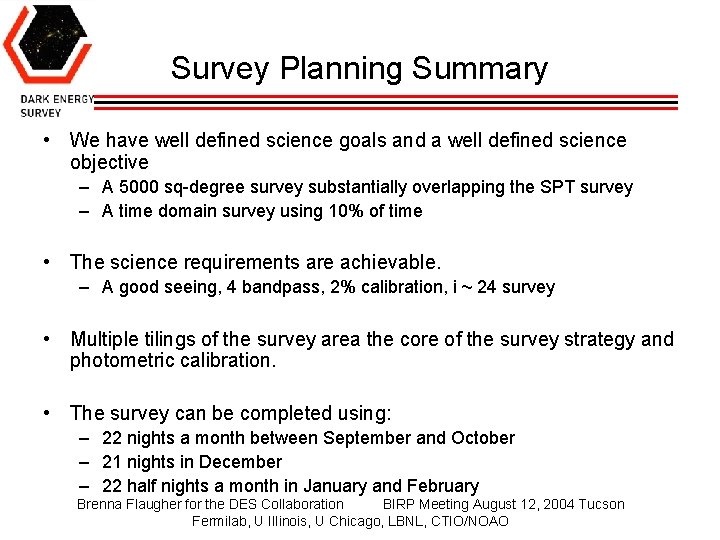
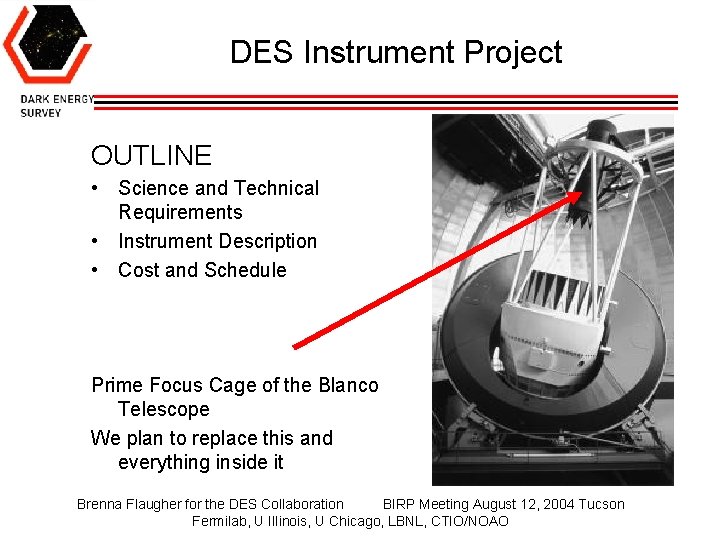
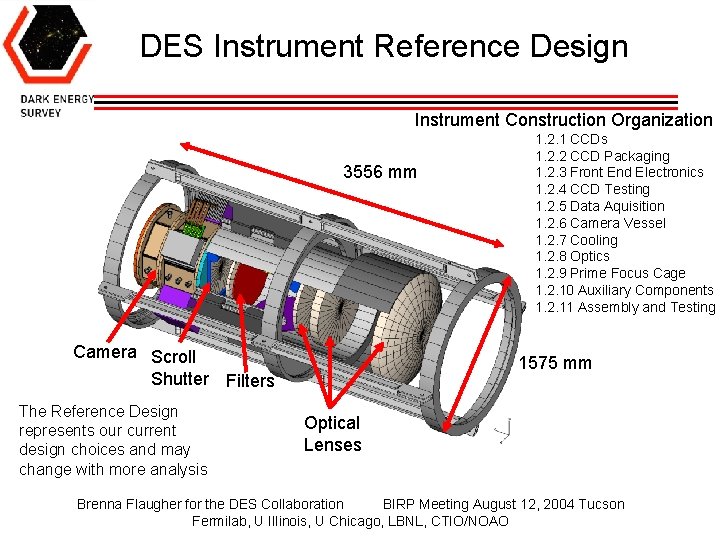
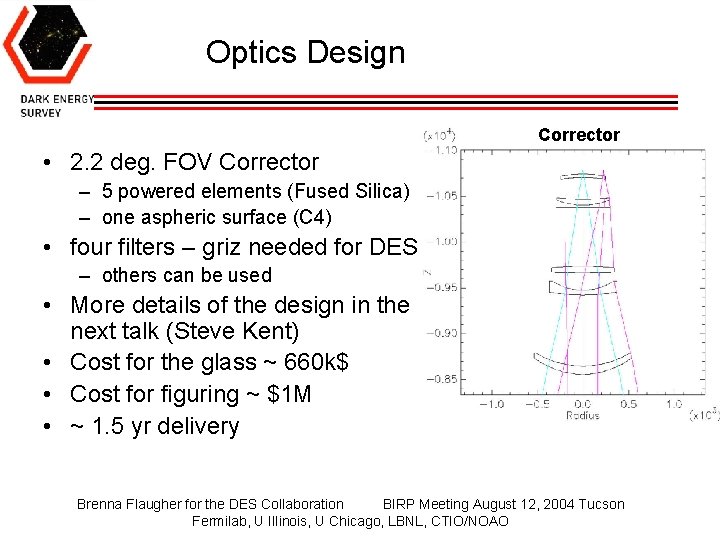
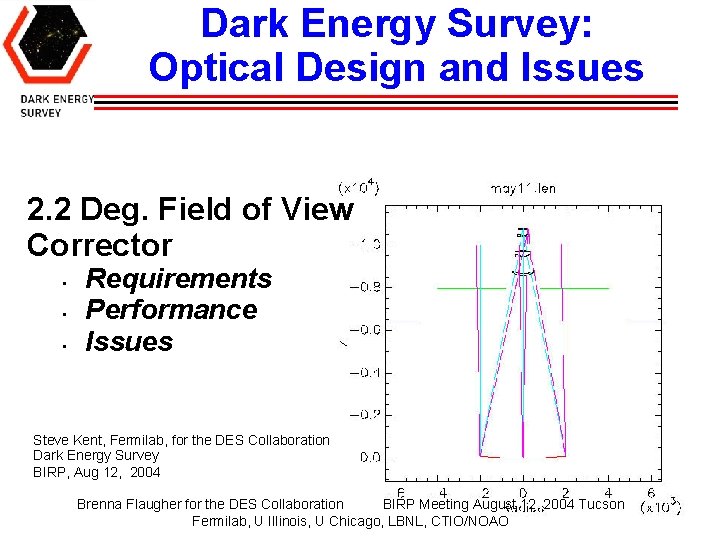
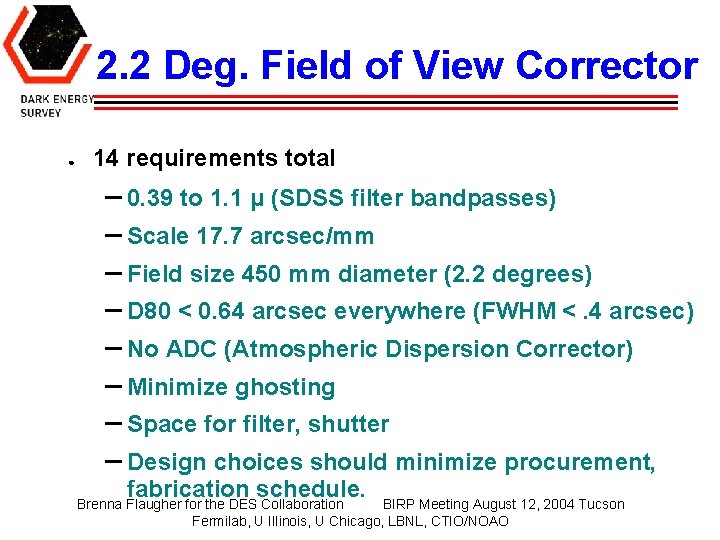
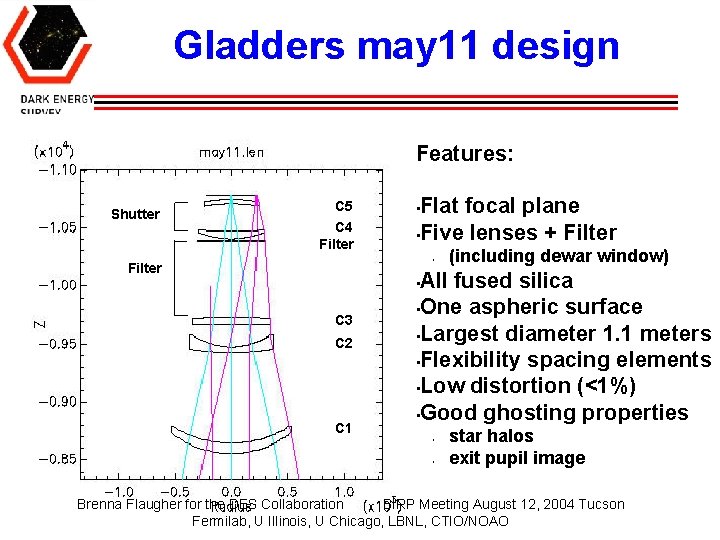
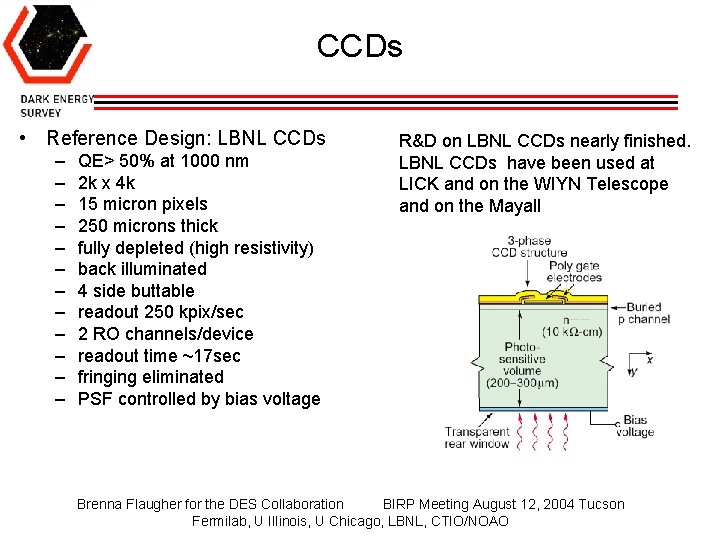
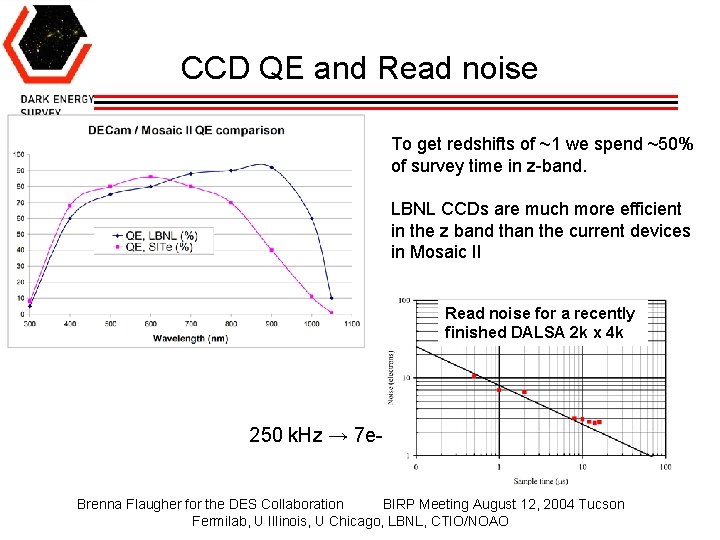
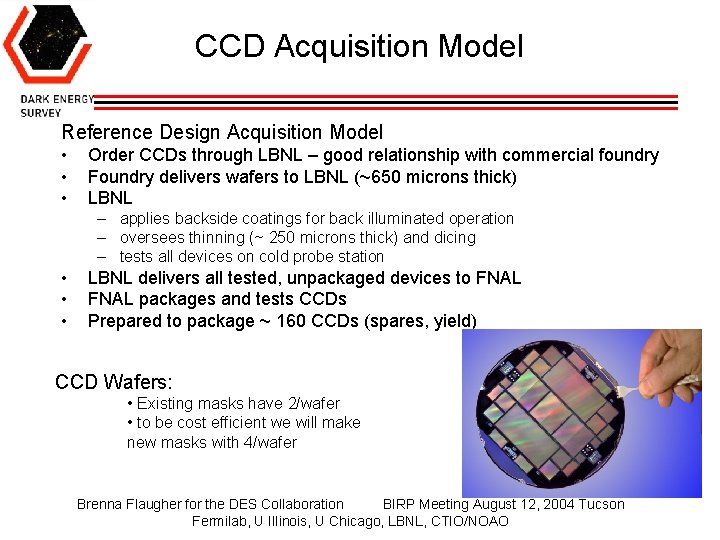
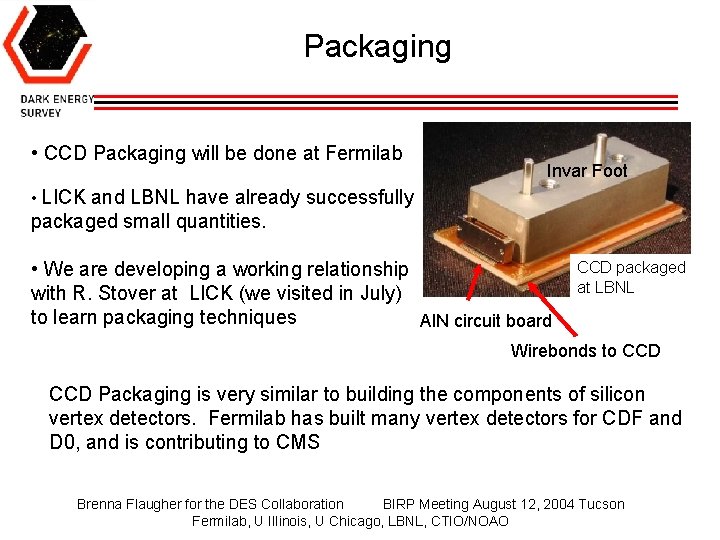
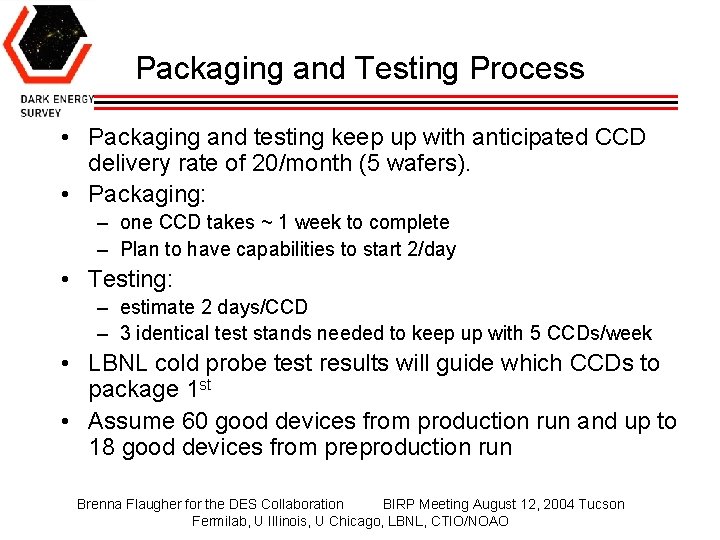
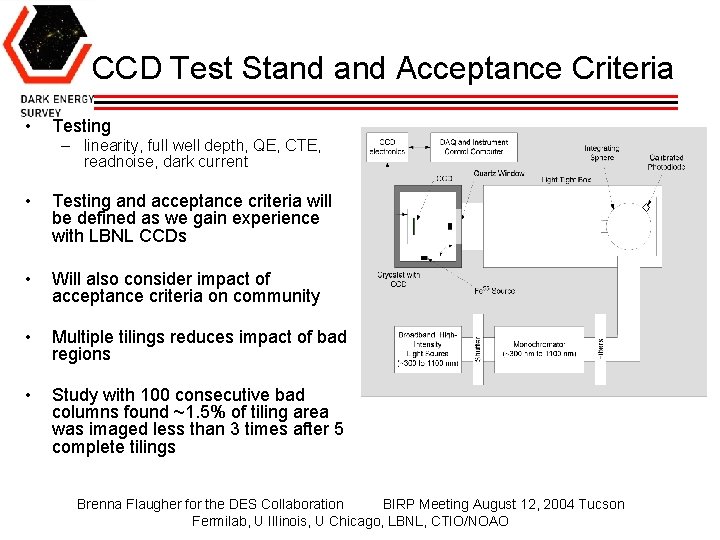
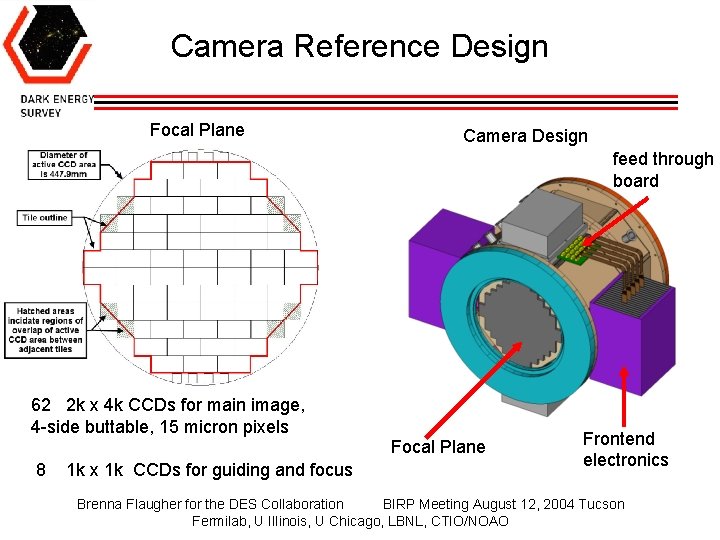
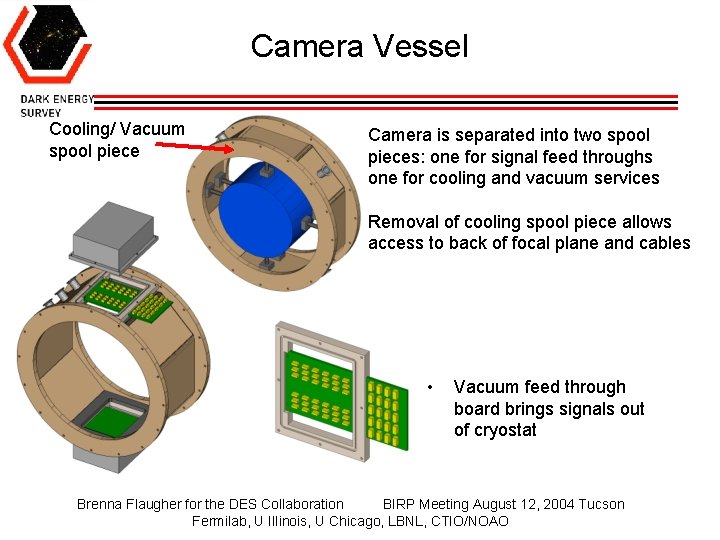
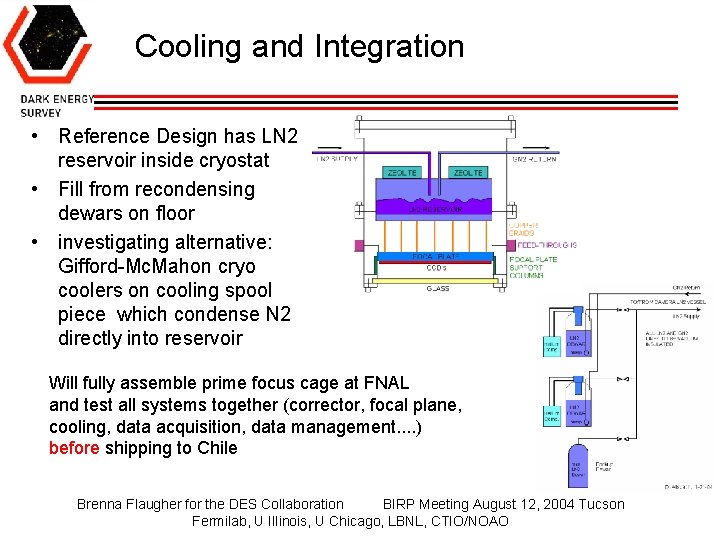
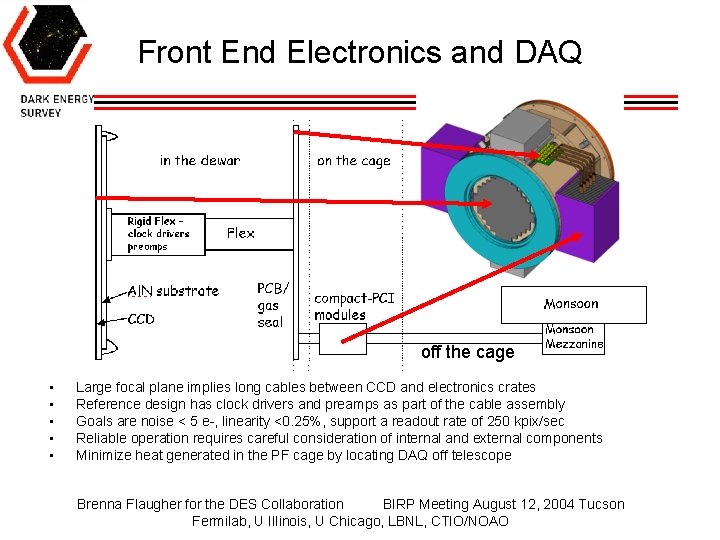
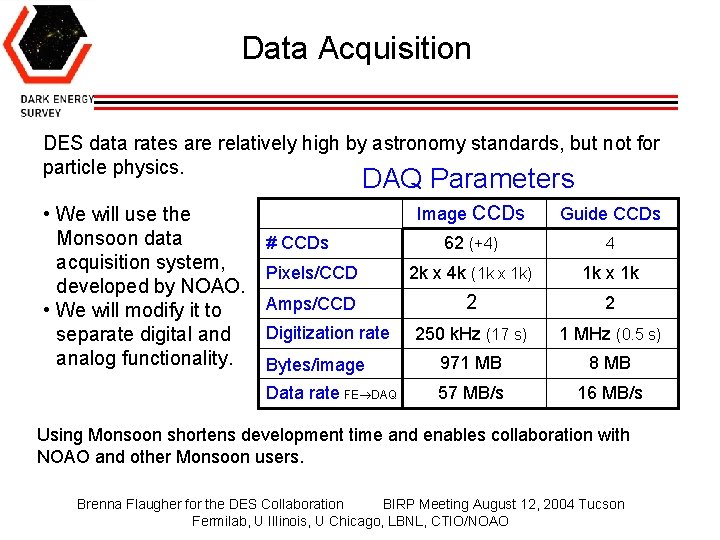
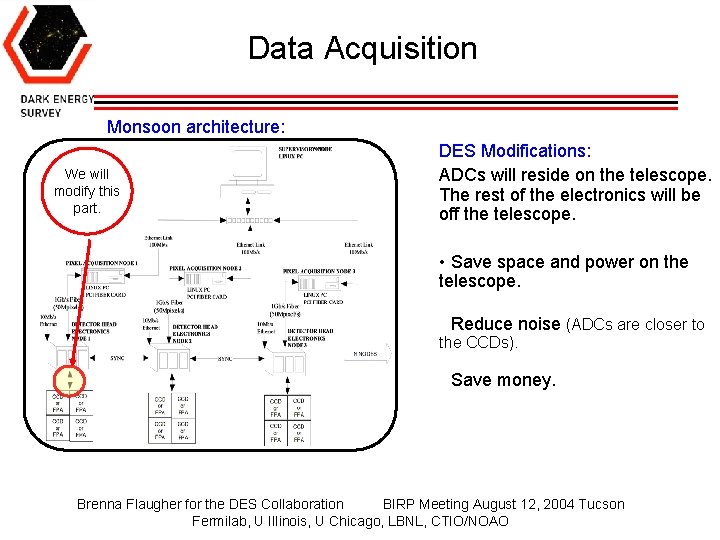
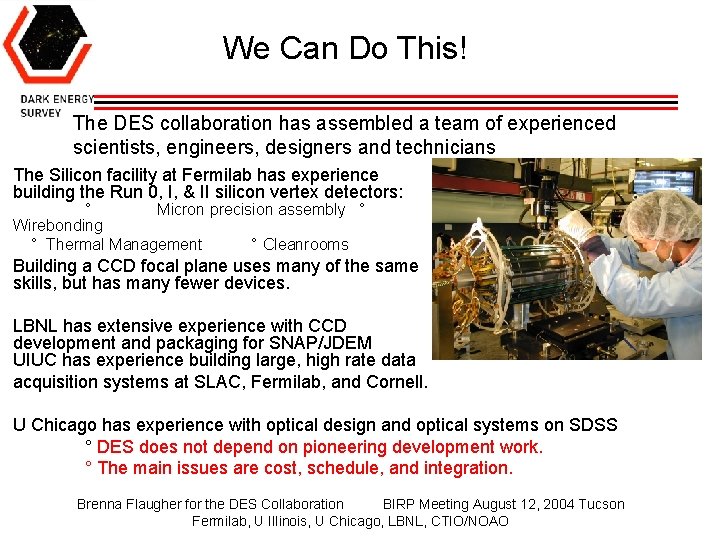
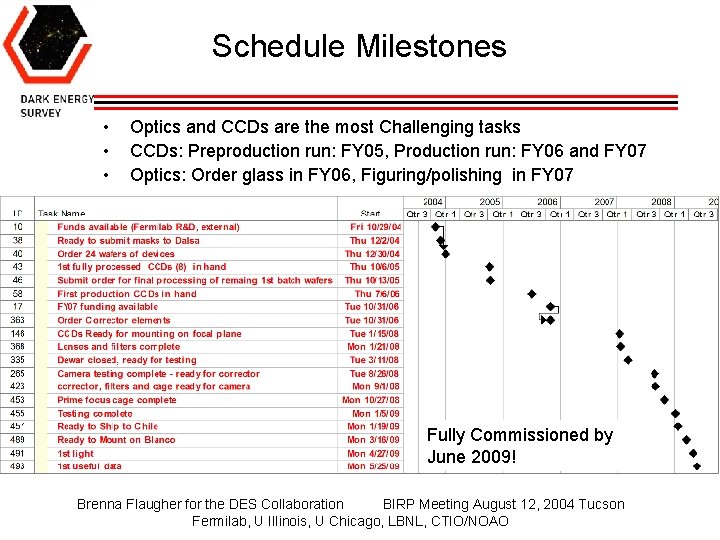
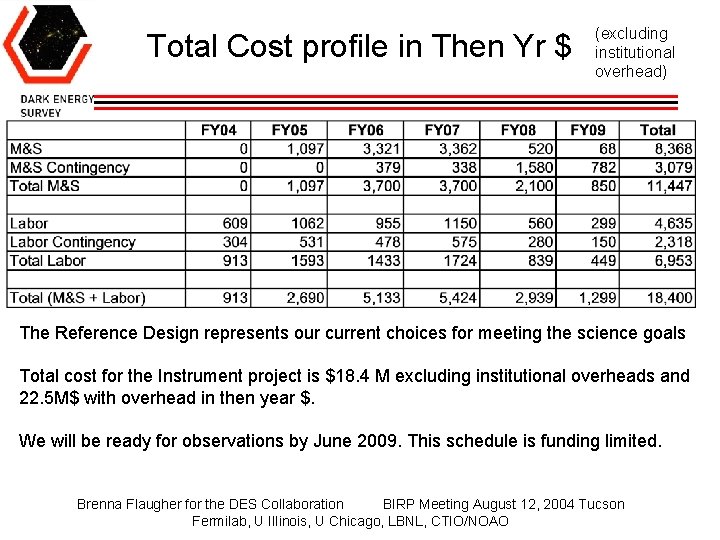
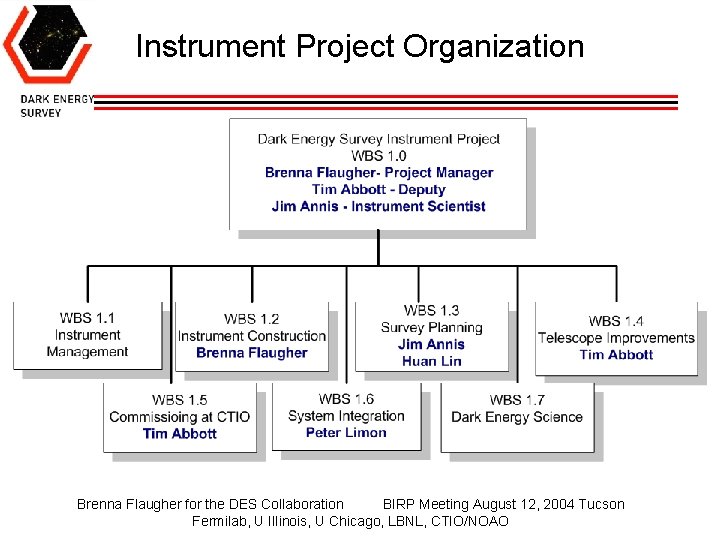
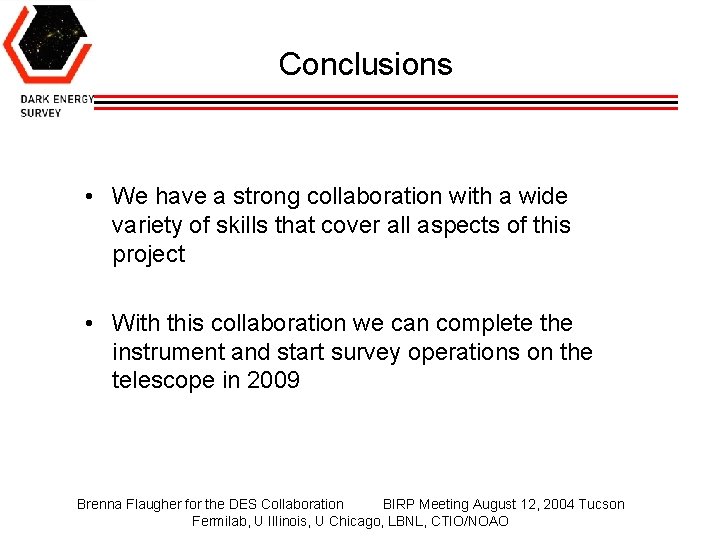
- Slides: 58
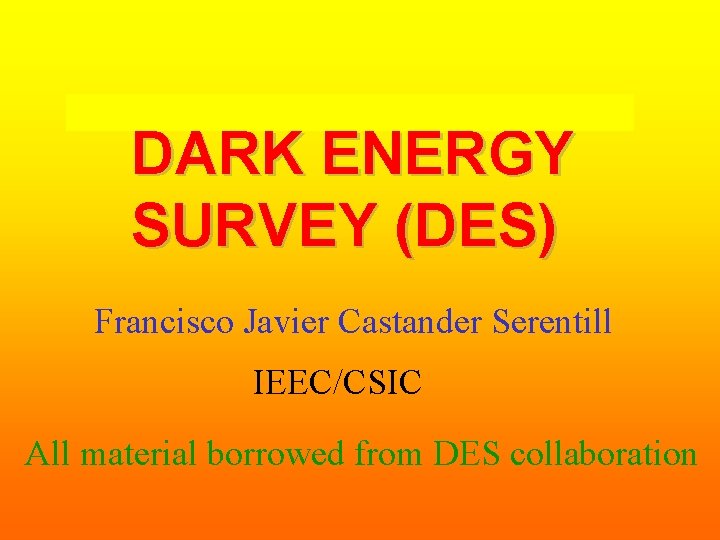
DARK ENERGY SURVEY (DES) Francisco Javier Castander Serentill IEEC/CSIC All material borrowed from DES collaboration
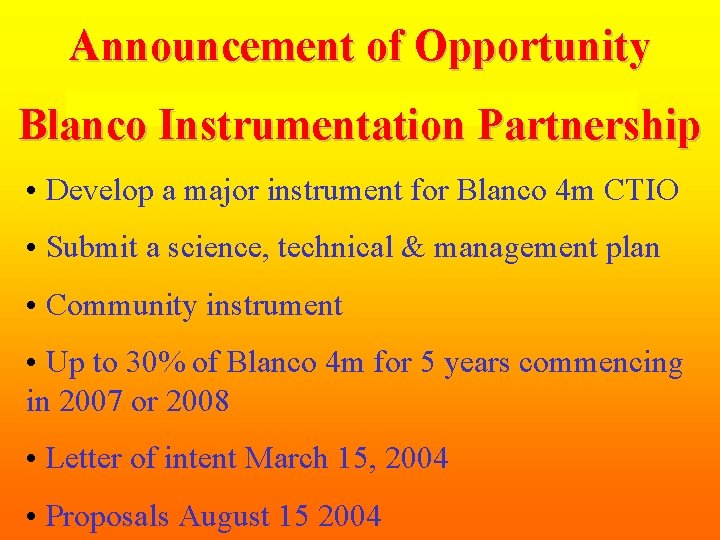
Announcement of Opportunity Blanco Instrumentation Partnership • Develop a major instrument for Blanco 4 m CTIO • Submit a science, technical & management plan • Community instrument • Up to 30% of Blanco 4 m for 5 years commencing in 2007 or 2008 • Letter of intent March 15, 2004 • Proposals August 15 2004
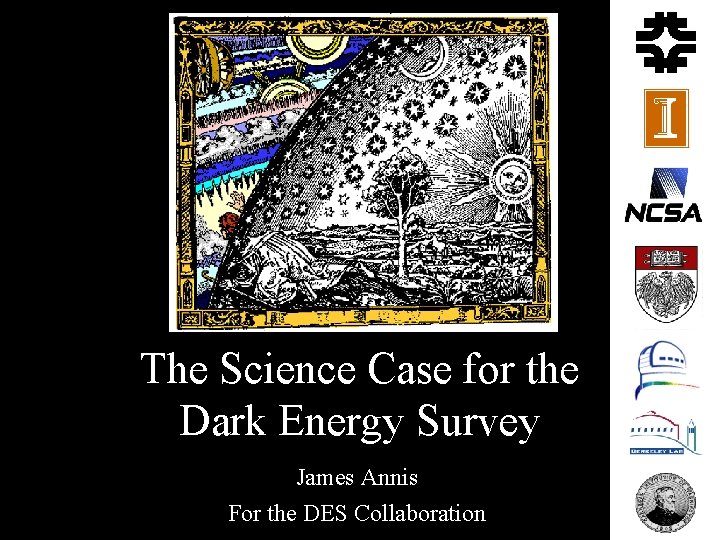
The Science Case for the Dark Energy Survey James Annis For the DES Collaboration
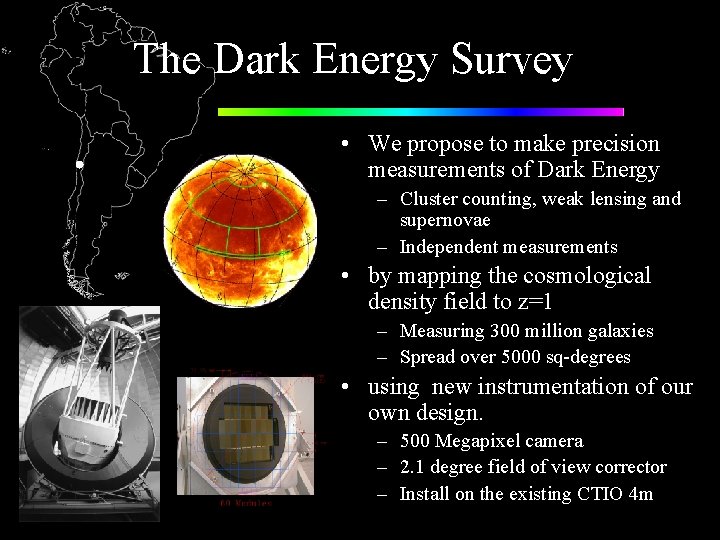
The Dark Energy Survey • We propose to make precision measurements of Dark Energy – Cluster counting, weak lensing and supernovae – Independent measurements • by mapping the cosmological density field to z=1 – Measuring 300 million galaxies – Spread over 5000 sq-degrees • using new instrumentation of our own design. – 500 Megapixel camera – 2. 1 degree field of view corrector – Install on the existing CTIO 4 m
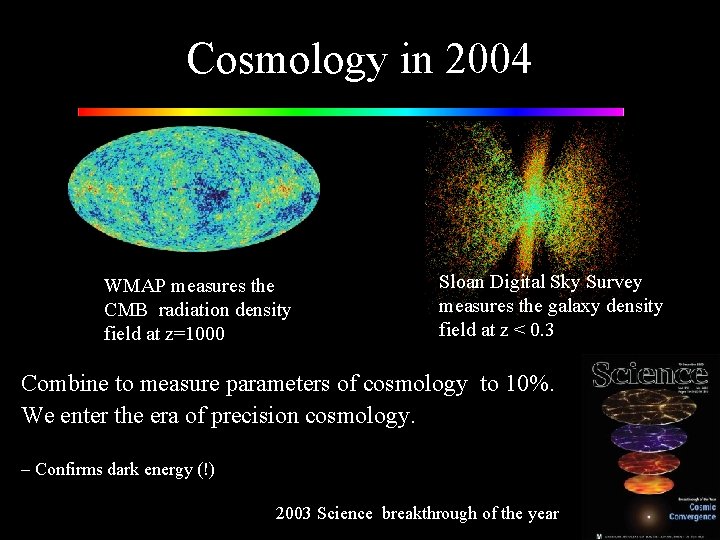
Cosmology in 2004 WMAP measures the CMB radiation density field at z=1000 Sloan Digital Sky Survey measures the galaxy density field at z < 0. 3 Combine to measure parameters of cosmology to 10%. We enter the era of precision cosmology. – Confirms dark energy (!) 2003 Science breakthrough of the year
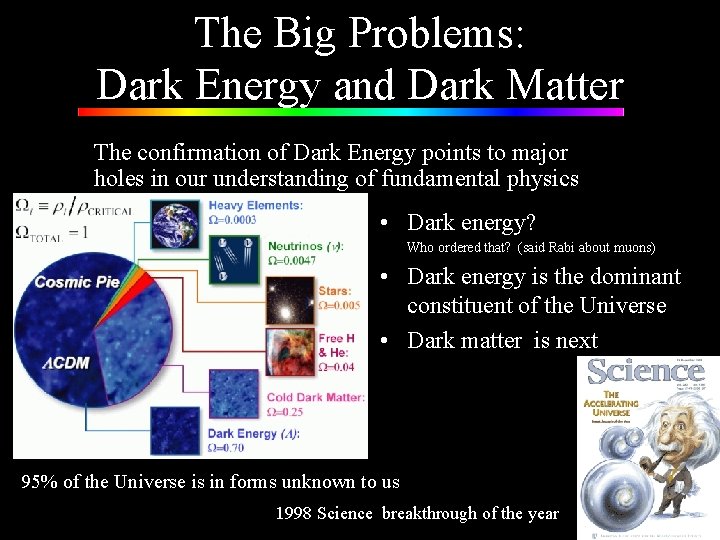
The Big Problems: Dark Energy and Dark Matter The confirmation of Dark Energy points to major holes in our understanding of fundamental physics • Dark energy? Who ordered that? (said Rabi about muons) • Dark energy is the dominant constituent of the Universe • Dark matter is next 95% of the Universe is in forms unknown to us 1998 Science breakthrough of the year
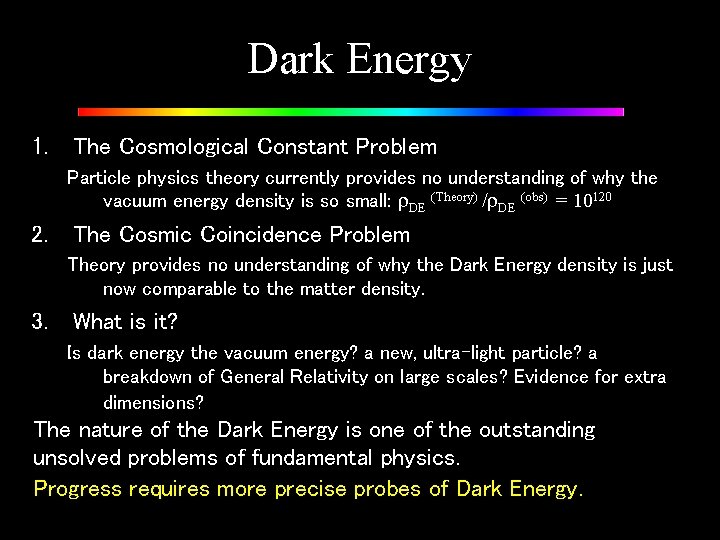
Dark Energy 1. The Cosmological Constant Problem Particle physics theory currently provides no understanding of why the vacuum energy density is so small: DE (Theory) / DE (obs) = 10120 2. The Cosmic Coincidence Problem Theory provides no understanding of why the Dark Energy density is just now comparable to the matter density. 3. What is it? Is dark energy the vacuum energy? a new, ultra-light particle? a breakdown of General Relativity on large scales? Evidence for extra dimensions? The nature of the Dark Energy is one of the outstanding unsolved problems of fundamental physics. Progress requires more precise probes of Dark Energy.
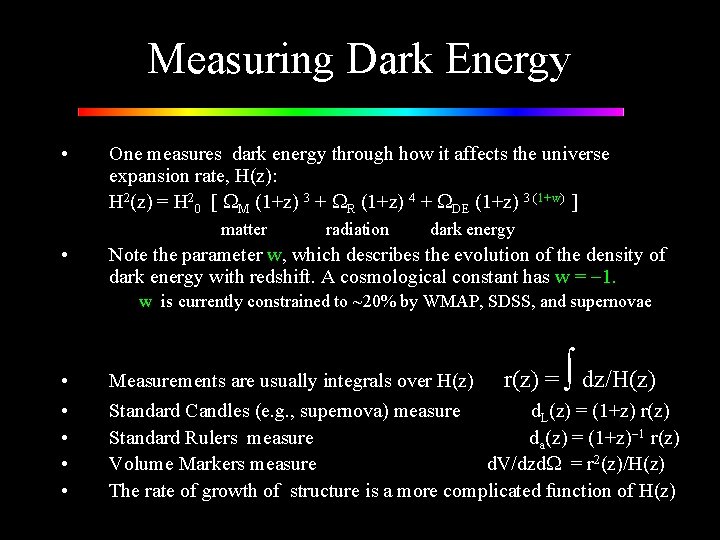
Measuring Dark Energy • One measures dark energy through how it affects the universe expansion rate, H(z): H 2(z) = H 20 [ M (1+z) 3 + R (1+z) 4 + DE (1+z) 3 (1+w) ] matter • radiation dark energy Note the parameter w, which describes the evolution of the density of dark energy with redshift. A cosmological constant has w = 1. w is currently constrained to ~20% by WMAP, SDSS, and supernovae • • • Measurements are usually integrals over H(z) r(z) = dz/H(z) Standard Candles (e. g. , supernova) measure d. L(z) = (1+z) r(z) Standard Rulers measure da(z) = (1+z) 1 r(z) Volume Markers measure d. V/dzd = r 2(z)/H(z) The rate of growth of structure is a more complicated function of H(z)
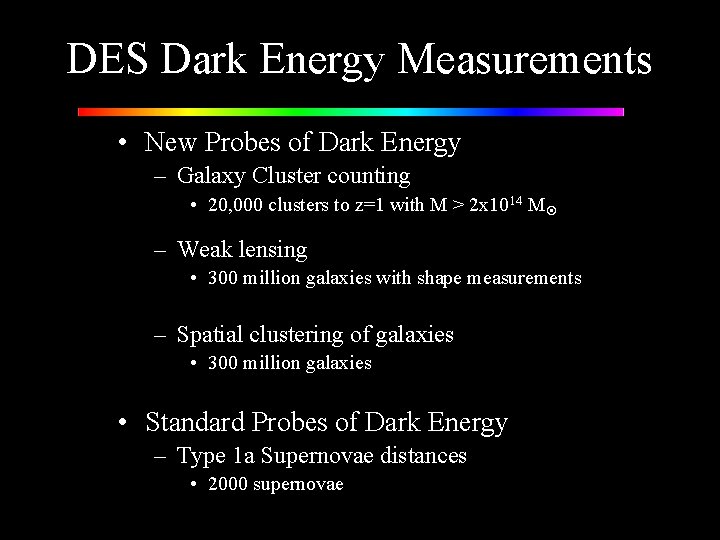
DES Dark Energy Measurements • New Probes of Dark Energy – Galaxy Cluster counting • 20, 000 clusters to z=1 with M > 2 x 1014 M – Weak lensing • 300 million galaxies with shape measurements – Spatial clustering of galaxies • 300 million galaxies • Standard Probes of Dark Energy – Type 1 a Supernovae distances • 2000 supernovae
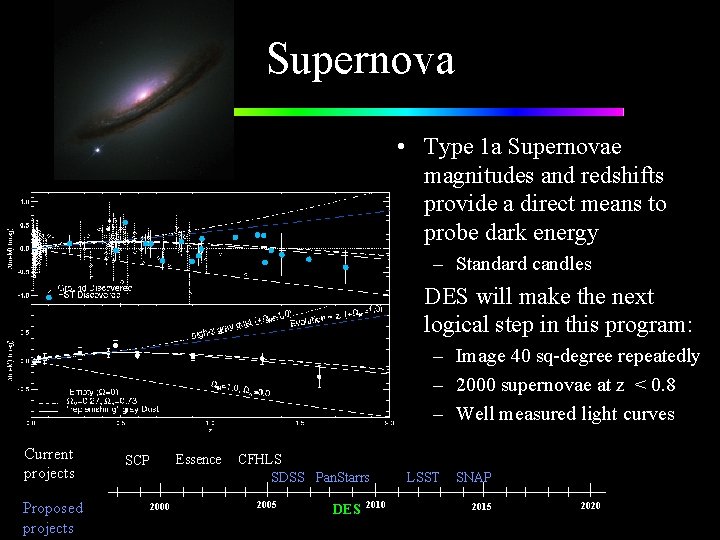
Supernova • Type 1 a Supernovae magnitudes and redshifts provide a direct means to probe dark energy – Standard candles • DES will make the next logical step in this program: – Image 40 sq-degree repeatedly – 2000 supernovae at z < 0. 8 – Well measured light curves Current projects Proposed projects SCP 2000 Essence CFHLS SDSS Pan. Starrs 2005 DES 2010 LSST SNAP 2015 2020
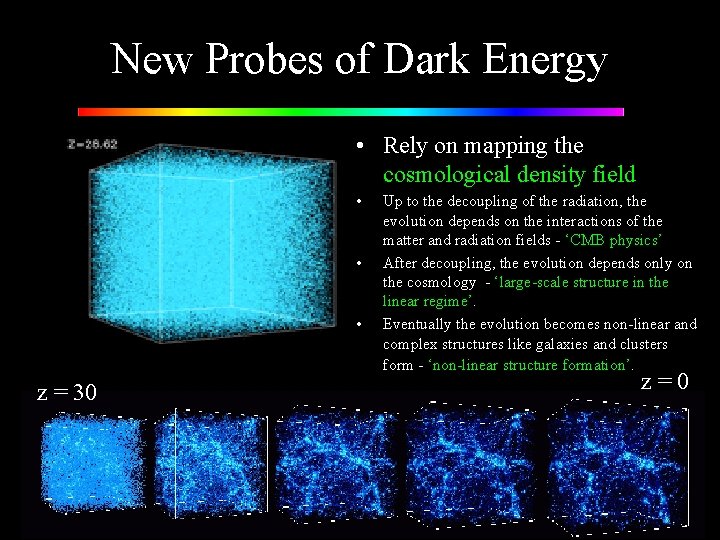
New Probes of Dark Energy • Rely on mapping the cosmological density field • • • z = 30 Up to the decoupling of the radiation, the evolution depends on the interactions of the matter and radiation fields - ‘CMB physics’ After decoupling, the evolution depends only on the cosmology - ‘large-scale structure in the linear regime’. Eventually the evolution becomes non-linear and complex structures like galaxies and clusters form - ‘non-linear structure formation’. z=0
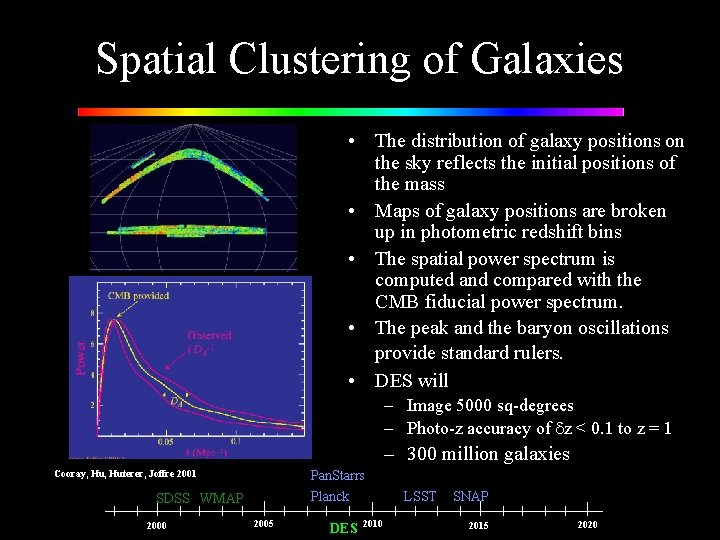
Spatial Clustering of Galaxies • The distribution of galaxy positions on the sky reflects the initial positions of the mass • Maps of galaxy positions are broken up in photometric redshift bins • The spatial power spectrum is computed and compared with the CMB fiducial power spectrum. • The peak and the baryon oscillations provide standard rulers. • DES will – Image 5000 sq-degrees – Photo-z accuracy of z < 0. 1 to z = 1 – 300 million galaxies Pan. Starrs Planck Cooray, Huterer, Joffre 2001 SDSS WMAP 2000 2005 DES 2010 LSST SNAP 2015 2020
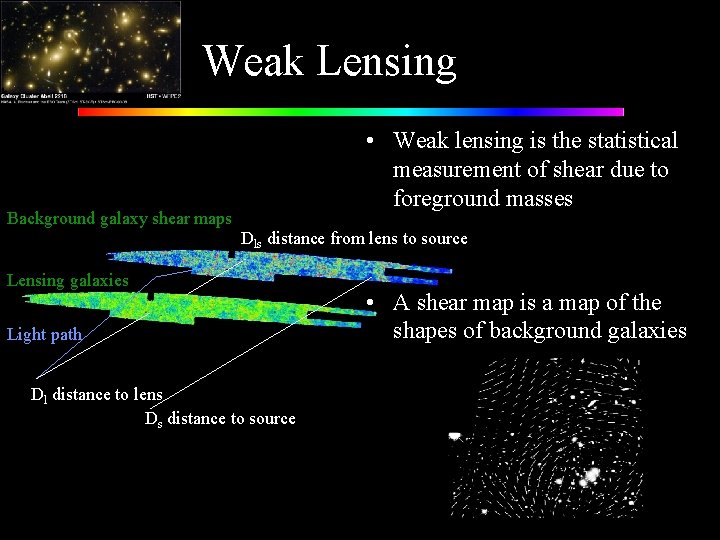
Weak Lensing • Weak lensing is the statistical measurement of shear due to foreground masses Background galaxy shear maps Dls distance from lens to source Lensing galaxies Light path Dl distance to lens Ds distance to source • A shear map is a map of the shapes of background galaxies
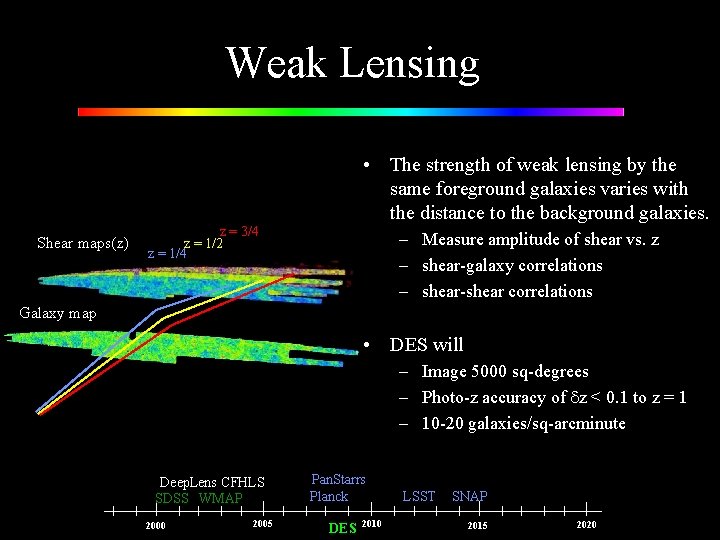
Weak Lensing • The strength of weak lensing by the same foreground galaxies varies with the distance to the background galaxies. Shear maps(z) z = 3/4 z = 1/2 z = 1/4 – Measure amplitude of shear vs. z – shear-galaxy correlations – shear-shear correlations Galaxy map • DES will – Image 5000 sq-degrees – Photo-z accuracy of z < 0. 1 to z = 1 – 10 -20 galaxies/sq-arcminute Deep. Lens CFHLS SDSS WMAP 2000 2005 Pan. Starrs Planck DES 2010 LSST SNAP 2015 2020
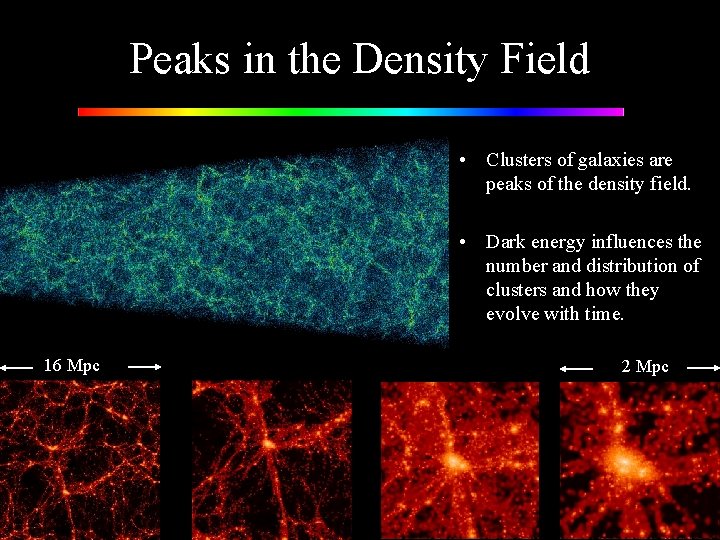
Peaks in the Density Field • Clusters of galaxies are peaks of the density field. • Dark energy influences the number and distribution of clusters and how they evolve with time. 16 Mpc 2 Mpc
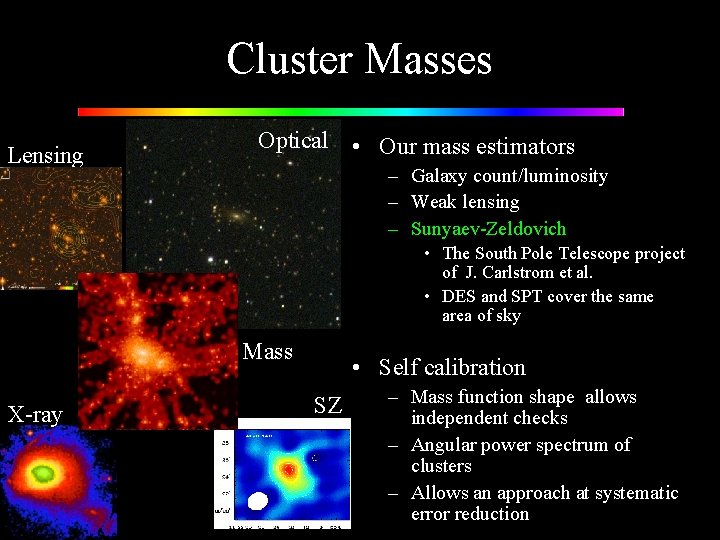
Cluster Masses Lensing Optical • Our mass estimators – Galaxy count/luminosity – Weak lensing – Sunyaev-Zeldovich • The South Pole Telescope project of J. Carlstrom et al. • DES and SPT cover the same area of sky Mass X-ray • Self calibration SZ – Mass function shape allows independent checks – Angular power spectrum of clusters – Allows an approach at systematic error reduction
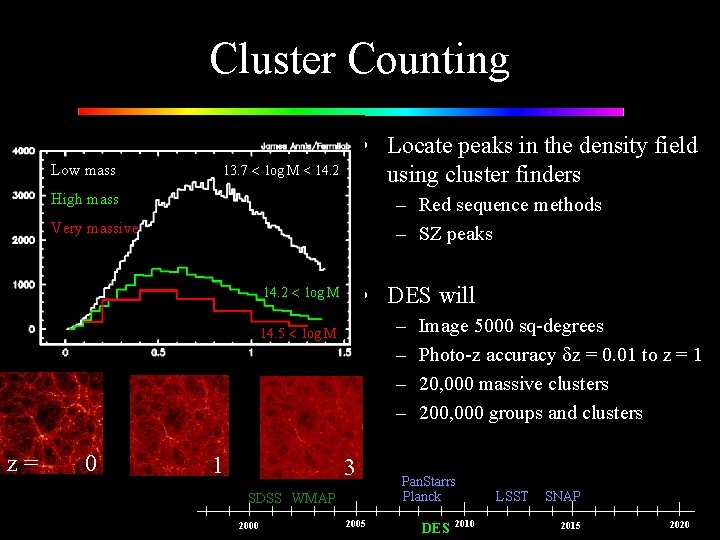
Cluster Counting Low mass • Locate peaks in the density field using cluster finders 13. 7 log M < 14. 2 High mass N – Red sequence methods – SZ peaks Very massive • DES will 14. 2 log M – – 14. 5 log M z z= 0 1 3 SDSS WMAP 2000 2005 Image 5000 sq-degrees Photo-z accuracy z = 0. 01 to z = 1 20, 000 massive clusters 200, 000 groups and clusters Pan. Starrs Planck DES 2010 LSST SNAP 2015 2020
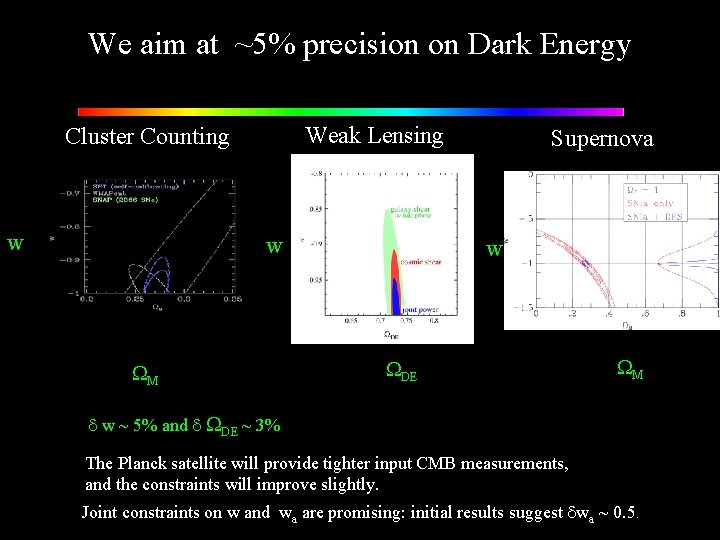
We aim at ~5% precision on Dark Energy Weak Lensing Cluster Counting w w M Supernova w DE M w ~ 5% and DE ~ 3% The Planck satellite will provide tighter input CMB measurements, and the constraints will improve slightly. Joint constraints on w and wa are promising: initial results suggest wa ~ 0. 5.
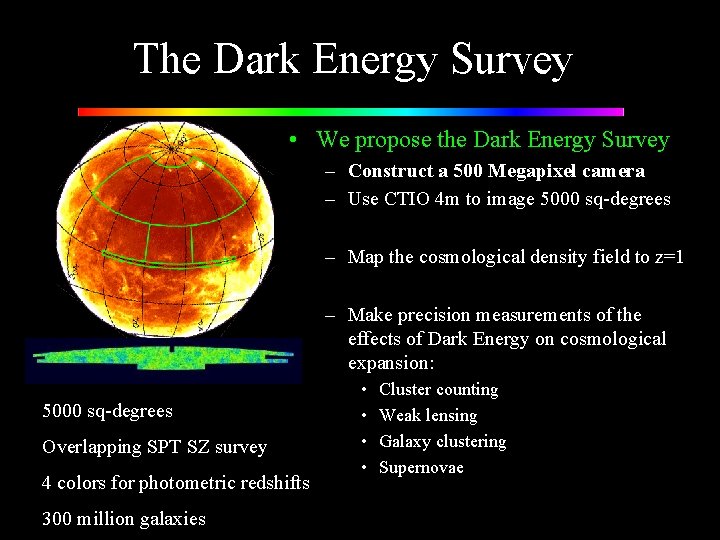
The Dark Energy Survey • We propose the Dark Energy Survey – Construct a 500 Megapixel camera – Use CTIO 4 m to image 5000 sq-degrees – Map the cosmological density field to z=1 – Make precision measurements of the effects of Dark Energy on cosmological expansion: 5000 sq-degrees Overlapping SPT SZ survey 4 colors for photometric redshifts 300 million galaxies • • Cluster counting Weak lensing Galaxy clustering Supernovae
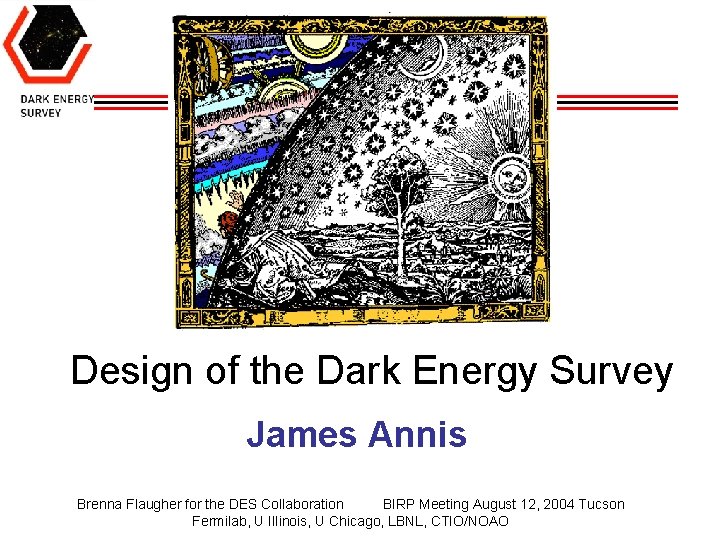
Design of the Dark Energy Survey James Annis Brenna Flaugher for the DES Collaboration BIRP Meeting August 12, 2004 Tucson Fermilab, U Illinois, U Chicago, LBNL, CTIO/NOAO
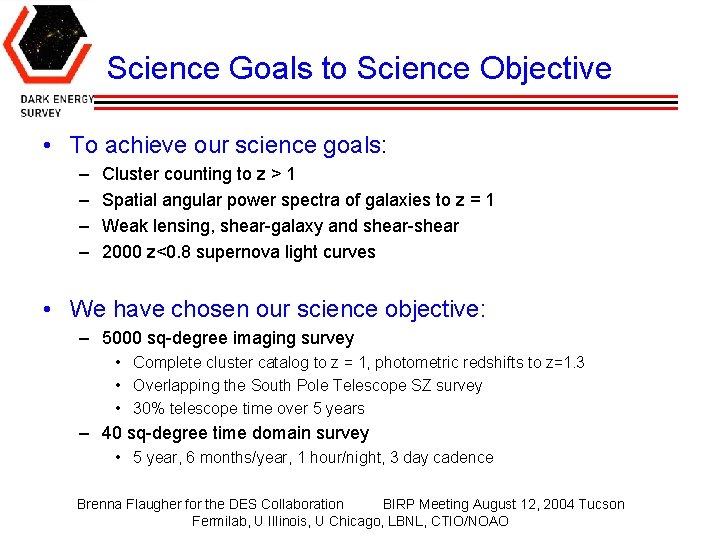
Science Goals to Science Objective • To achieve our science goals: – – Cluster counting to z > 1 Spatial angular power spectra of galaxies to z = 1 Weak lensing, shear-galaxy and shear-shear 2000 z<0. 8 supernova light curves • We have chosen our science objective: – 5000 sq-degree imaging survey • Complete cluster catalog to z = 1, photometric redshifts to z=1. 3 • Overlapping the South Pole Telescope SZ survey • 30% telescope time over 5 years – 40 sq-degree time domain survey • 5 year, 6 months/year, 1 hour/night, 3 day cadence Brenna Flaugher for the DES Collaboration BIRP Meeting August 12, 2004 Tucson Fermilab, U Illinois, U Chicago, LBNL, CTIO/NOAO
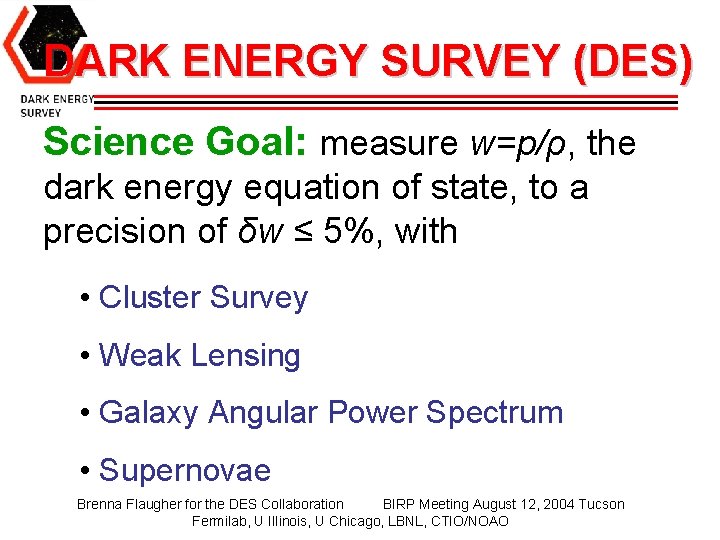
DARK ENERGY SURVEY (DES) Science Goal: measure w=p/ρ, the dark energy equation of state, to a precision of δw ≤ 5%, with • Cluster Survey • Weak Lensing • Galaxy Angular Power Spectrum • Supernovae Brenna Flaugher for the DES Collaboration BIRP Meeting August 12, 2004 Tucson Fermilab, U Illinois, U Chicago, LBNL, CTIO/NOAO
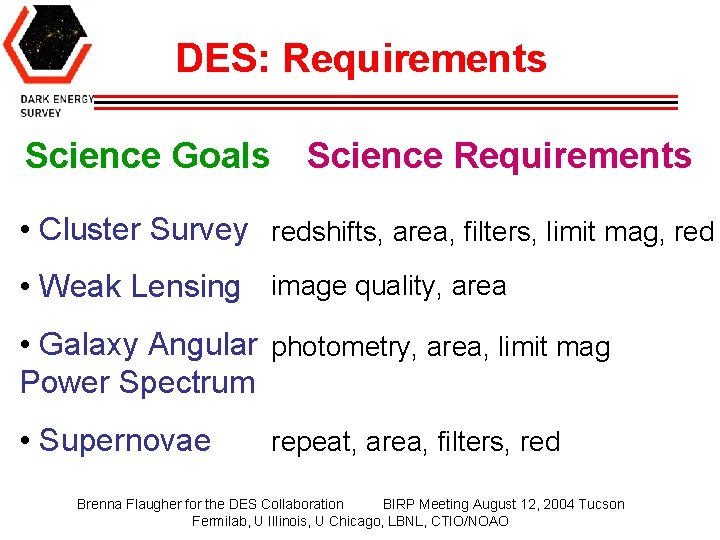
DES: Requirements Science Goals Science Requirements • Cluster Survey redshifts, area, filters, limit mag, red • Weak Lensing image quality, area • Galaxy Angular photometry, area, limit mag Power Spectrum • Supernovae repeat, area, filters, red Brenna Flaugher for the DES Collaboration BIRP Meeting August 12, 2004 Tucson Fermilab, U Illinois, U Chicago, LBNL, CTIO/NOAO
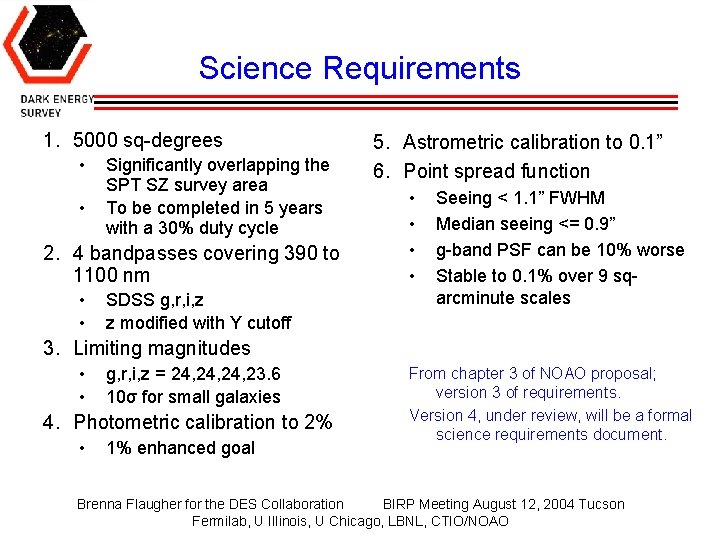
Science Requirements 1. 5000 sq-degrees • • Significantly overlapping the SPT SZ survey area To be completed in 5 years with a 30% duty cycle 2. 4 bandpasses covering 390 to 1100 nm • • SDSS g, r, i, z z modified with Y cutoff 5. Astrometric calibration to 0. 1” 6. Point spread function • • Seeing < 1. 1” FWHM Median seeing <= 0. 9” g-band PSF can be 10% worse Stable to 0. 1% over 9 sqarcminute scales 3. Limiting magnitudes • • g, r, i, z = 24, 24, 23. 6 10σ for small galaxies 4. Photometric calibration to 2% • 1% enhanced goal From chapter 3 of NOAO proposal; version 3 of requirements. Version 4, under review, will be a formal science requirements document. Brenna Flaugher for the DES Collaboration BIRP Meeting August 12, 2004 Tucson Fermilab, U Illinois, U Chicago, LBNL, CTIO/NOAO
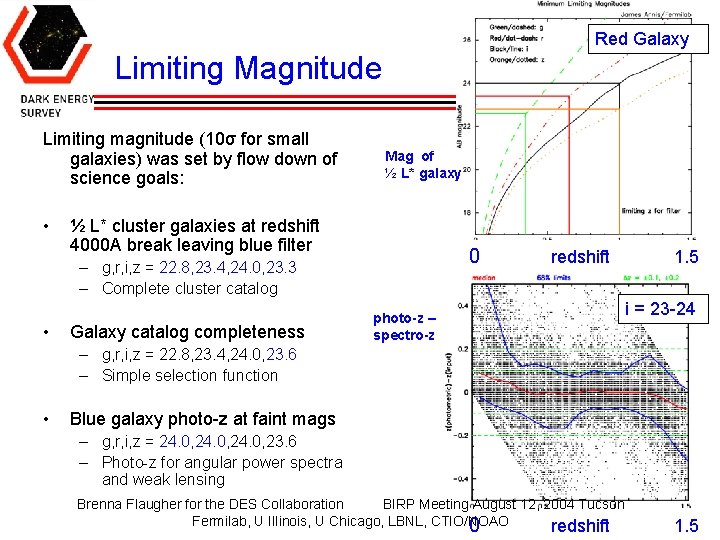
Red Galaxy Limiting Magnitude Limiting magnitude (10σ for small galaxies) was set by flow down of science goals: • Mag of ½ L* galaxy ½ L* cluster galaxies at redshift 4000 A break leaving blue filter 0 – g, r, i, z = 22. 8, 23. 4, 24. 0, 23. 3 – Complete cluster catalog • Galaxy catalog completeness redshift photo-z – spectro-z 1. 5 i = 23 -24 – g, r, i, z = 22. 8, 23. 4, 24. 0, 23. 6 – Simple selection function • Blue galaxy photo-z at faint mags – g, r, i, z = 24. 0, 23. 6 – Photo-z for angular power spectra and weak lensing Brenna Flaugher for the DES Collaboration BIRP Meeting August 12, 2004 Tucson Fermilab, U Illinois, U Chicago, LBNL, CTIO/NOAO 0 redshift 1. 5
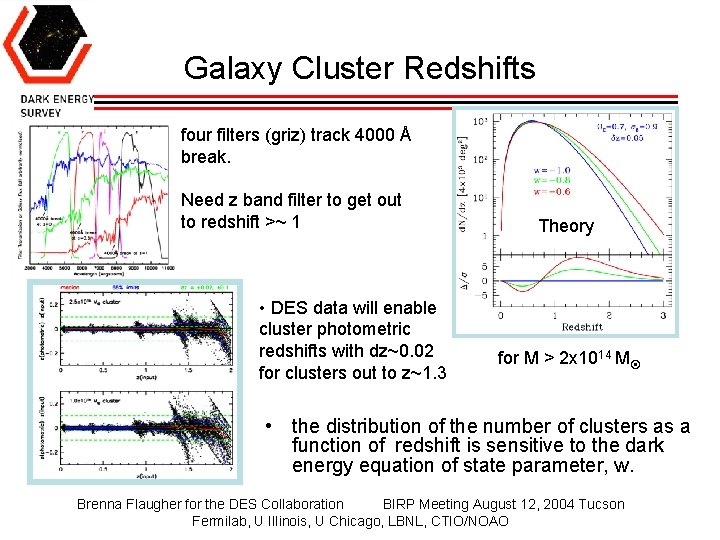
Galaxy Cluster Redshifts four filters (griz) track 4000 Å break. Need z band filter to get out to redshift >~ 1 • DES data will enable cluster photometric redshifts with dz~0. 02 for clusters out to z~1. 3 Theory for M > 2 x 1014 M • the distribution of the number of clusters as a function of redshift is sensitive to the dark energy equation of state parameter, w. Brenna Flaugher for the DES Collaboration BIRP Meeting August 12, 2004 Tucson Fermilab, U Illinois, U Chicago, LBNL, CTIO/NOAO
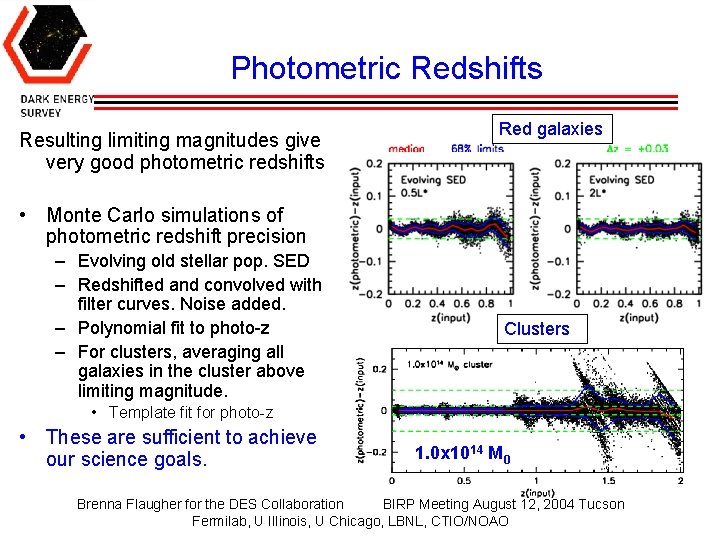
Photometric Redshifts Red galaxies Resulting limiting magnitudes give very good photometric redshifts • Monte Carlo simulations of photometric redshift precision – Evolving old stellar pop. SED – Redshifted and convolved with filter curves. Noise added. – Polynomial fit to photo-z – For clusters, averaging all galaxies in the cluster above limiting magnitude. ½ L* 2 L* Clusters • Template fit for photo-z • These are sufficient to achieve our science goals. 1. 0 x 1014 M 0 Brenna Flaugher for the DES Collaboration BIRP Meeting August 12, 2004 Tucson Fermilab, U Illinois, U Chicago, LBNL, CTIO/NOAO
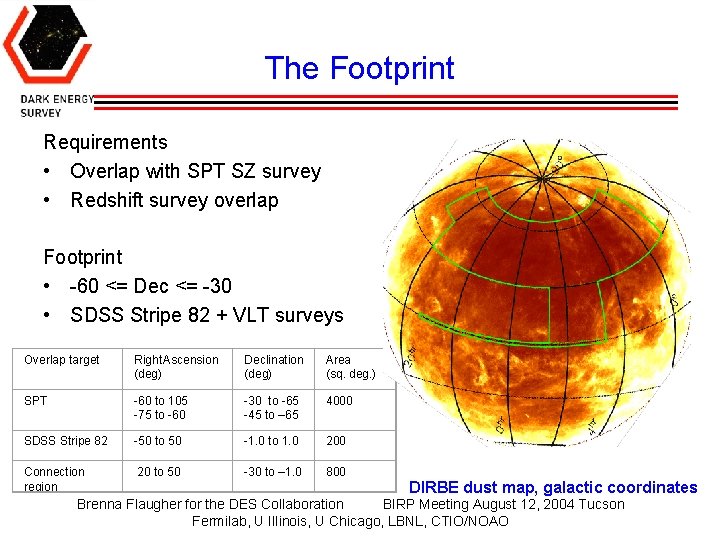
The Footprint Requirements • Overlap with SPT SZ survey • Redshift survey overlap Footprint • -60 <= Dec <= -30 • SDSS Stripe 82 + VLT surveys Overlap target Right Ascension (deg) Declination (deg) Area (sq. deg. ) SPT -60 to 105 -75 to -60 -30 to -65 -45 to – 65 4000 SDSS Stripe 82 -50 to 50 -1. 0 to 1. 0 200 Connection region 20 to 50 -30 to – 1. 0 800 DIRBE dust map, galactic coordinates Brenna Flaugher for the DES Collaboration BIRP Meeting August 12, 2004 Tucson Fermilab, U Illinois, U Chicago, LBNL, CTIO/NOAO
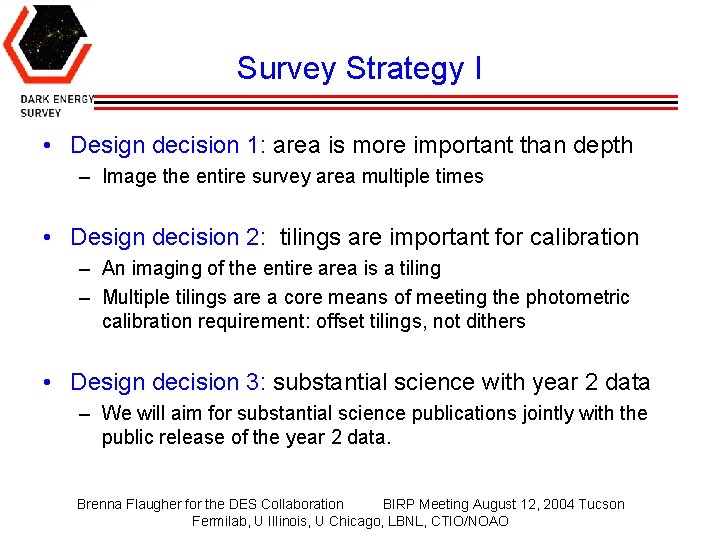
Survey Strategy I • Design decision 1: area is more important than depth – Image the entire survey area multiple times • Design decision 2: tilings are important for calibration – An imaging of the entire area is a tiling – Multiple tilings are a core means of meeting the photometric calibration requirement: offset tilings, not dithers • Design decision 3: substantial science with year 2 data – We will aim for substantial science publications jointly with the public release of the year 2 data. Brenna Flaugher for the DES Collaboration BIRP Meeting August 12, 2004 Tucson Fermilab, U Illinois, U Chicago, LBNL, CTIO/NOAO
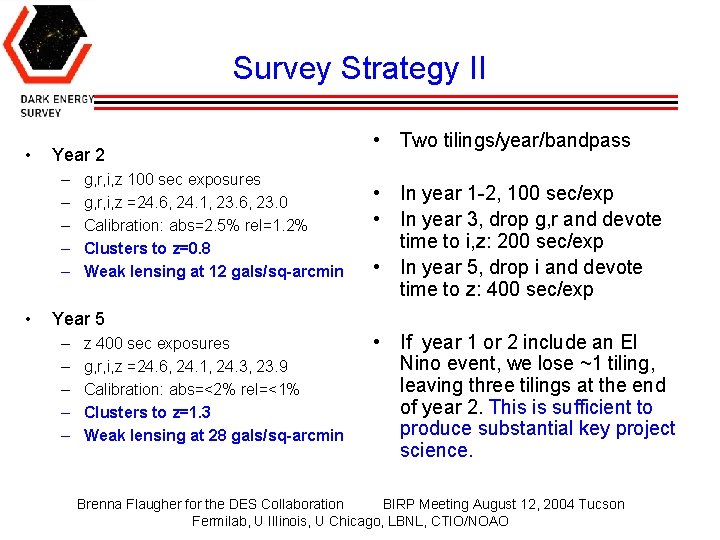
Survey Strategy II • Year 2 – – – • g, r, i, z 100 sec exposures g, r, i, z =24. 6, 24. 1, 23. 6, 23. 0 Calibration: abs=2. 5% rel=1. 2% Clusters to z=0. 8 Weak lensing at 12 gals/sq-arcmin • Two tilings/year/bandpass • In year 1 -2, 100 sec/exp • In year 3, drop g, r and devote time to i, z: 200 sec/exp • In year 5, drop i and devote time to z: 400 sec/exp Year 5 – – – z 400 sec exposures g, r, i, z =24. 6, 24. 1, 24. 3, 23. 9 Calibration: abs=<2% rel=<1% Clusters to z=1. 3 Weak lensing at 28 gals/sq-arcmin • If year 1 or 2 include an El Nino event, we lose ~1 tiling, leaving three tilings at the end of year 2. This is sufficient to produce substantial key project science. Brenna Flaugher for the DES Collaboration BIRP Meeting August 12, 2004 Tucson Fermilab, U Illinois, U Chicago, LBNL, CTIO/NOAO
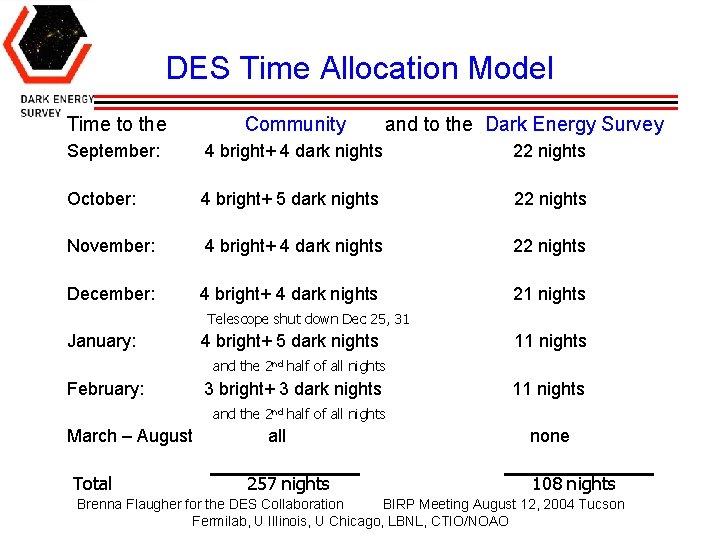
DES Time Allocation Model Time to the Community and to the Dark Energy Survey September: 4 bright+ 4 dark nights 22 nights October: 4 bright+ 5 dark nights 22 nights November: 4 bright+ 4 dark nights 22 nights December: 4 bright+ 4 dark nights 21 nights Telescope shut down Dec 25, 31 January: 4 bright+ 5 dark nights 11 nights and the 2 nd half of all nights February: 3 bright+ 3 dark nights 11 nights and the 2 nd half of all nights March – August Total all 257 nights none 108 nights Brenna Flaugher for the DES Collaboration BIRP Meeting August 12, 2004 Tucson Fermilab, U Illinois, U Chicago, LBNL, CTIO/NOAO
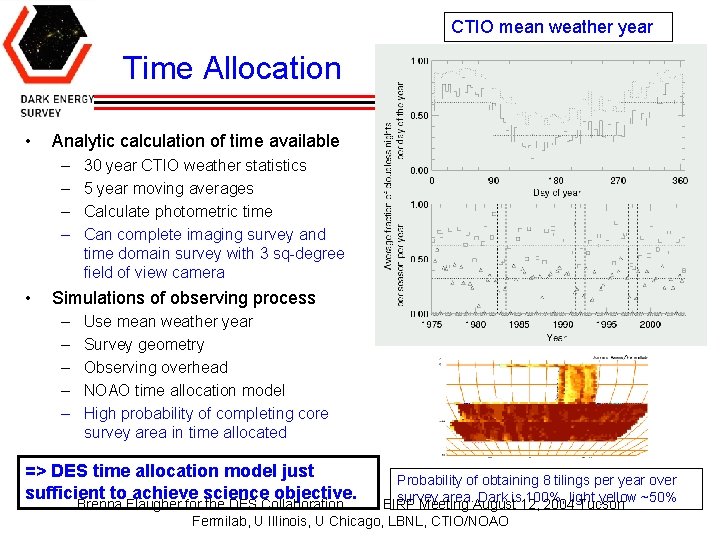
CTIO mean weather year Time Allocation • Analytic calculation of time available – – • 30 year CTIO weather statistics 5 year moving averages Calculate photometric time Can complete imaging survey and time domain survey with 3 sq-degree field of view camera Simulations of observing process – – – Use mean weather year Survey geometry Observing overhead NOAO time allocation model High probability of completing core survey area in time allocated => DES time allocation model just sufficient to achieve science objective. Probability of obtaining 8 tilings per year over survey area. August Dark is 12, 100%, yellow ~50% BIRP Meeting 2004 light Tucson Brenna Flaugher for the DES Collaboration Fermilab, U Illinois, U Chicago, LBNL, CTIO/NOAO
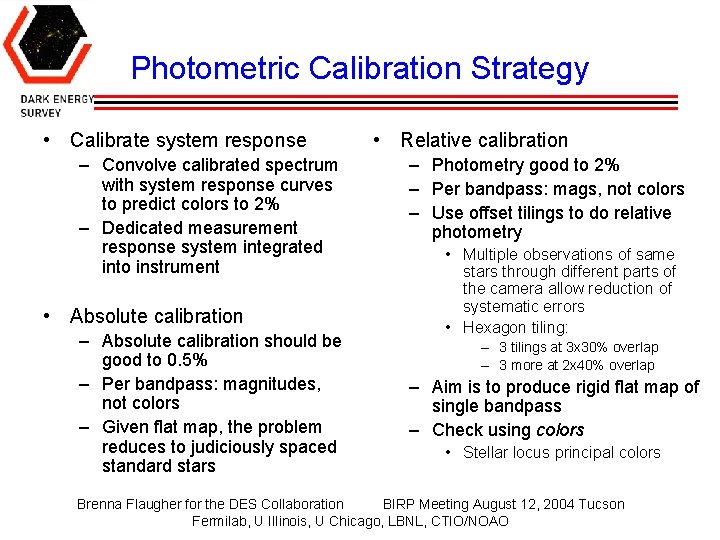
Photometric Calibration Strategy • Calibrate system response – Convolve calibrated spectrum with system response curves to predict colors to 2% – Dedicated measurement response system integrated into instrument • Absolute calibration – Absolute calibration should be good to 0. 5% – Per bandpass: magnitudes, not colors – Given flat map, the problem reduces to judiciously spaced standard stars • Relative calibration – Photometry good to 2% – Per bandpass: mags, not colors – Use offset tilings to do relative photometry • Multiple observations of same stars through different parts of the camera allow reduction of systematic errors • Hexagon tiling: – 3 tilings at 3 x 30% overlap – 3 more at 2 x 40% overlap – Aim is to produce rigid flat map of single bandpass – Check using colors • Stellar locus principal colors Brenna Flaugher for the DES Collaboration BIRP Meeting August 12, 2004 Tucson Fermilab, U Illinois, U Chicago, LBNL, CTIO/NOAO
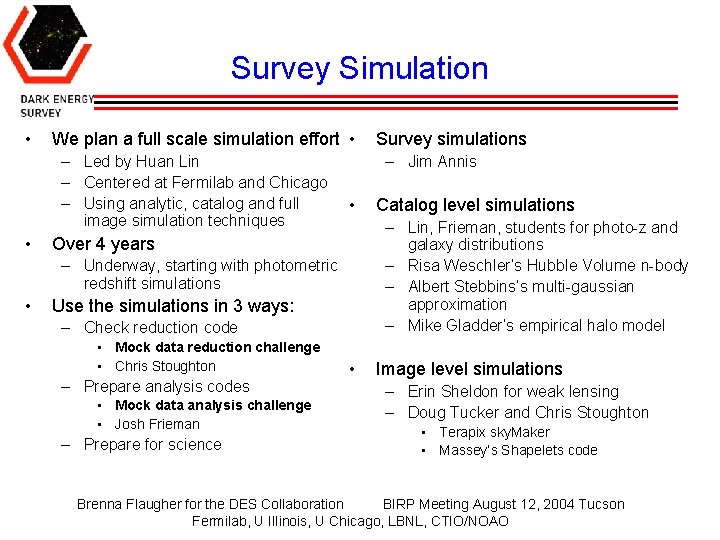
Survey Simulation • We plan a full scale simulation effort • – Led by Huan Lin – Centered at Fermilab and Chicago – Using analytic, catalog and full image simulation techniques • – Jim Annis • Over 4 years Use the simulations in 3 ways: – Check reduction code • Mock data reduction challenge • Chris Stoughton – Prepare analysis codes • Mock data analysis challenge • Josh Frieman – Prepare for science Catalog level simulations – Lin, Frieman, students for photo-z and galaxy distributions – Risa Weschler’s Hubble Volume n-body – Albert Stebbins’s multi-gaussian approximation – Mike Gladder’s empirical halo model – Underway, starting with photometric redshift simulations • Survey simulations • Image level simulations – Erin Sheldon for weak lensing – Doug Tucker and Chris Stoughton • Terapix sky. Maker • Massey’s Shapelets code Brenna Flaugher for the DES Collaboration BIRP Meeting August 12, 2004 Tucson Fermilab, U Illinois, U Chicago, LBNL, CTIO/NOAO
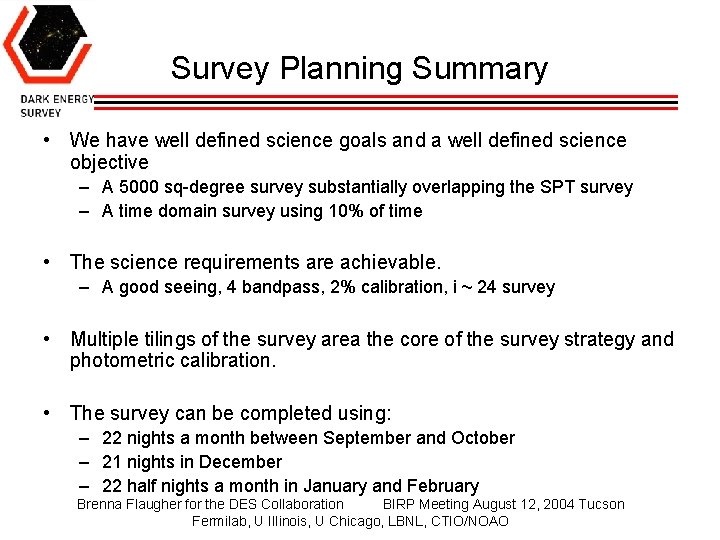
Survey Planning Summary • We have well defined science goals and a well defined science objective – A 5000 sq-degree survey substantially overlapping the SPT survey – A time domain survey using 10% of time • The science requirements are achievable. – A good seeing, 4 bandpass, 2% calibration, i ~ 24 survey • Multiple tilings of the survey area the core of the survey strategy and photometric calibration. • The survey can be completed using: – 22 nights a month between September and October – 21 nights in December – 22 half nights a month in January and February Brenna Flaugher for the DES Collaboration BIRP Meeting August 12, 2004 Tucson Fermilab, U Illinois, U Chicago, LBNL, CTIO/NOAO
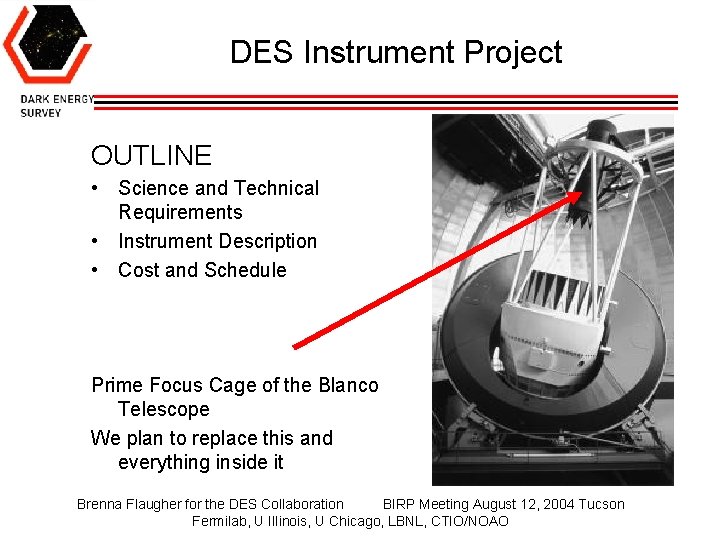
DES Instrument Project OUTLINE • Science and Technical Requirements • Instrument Description • Cost and Schedule Prime Focus Cage of the Blanco Telescope We plan to replace this and everything inside it Brenna Flaugher for the DES Collaboration BIRP Meeting August 12, 2004 Tucson Fermilab, U Illinois, U Chicago, LBNL, CTIO/NOAO
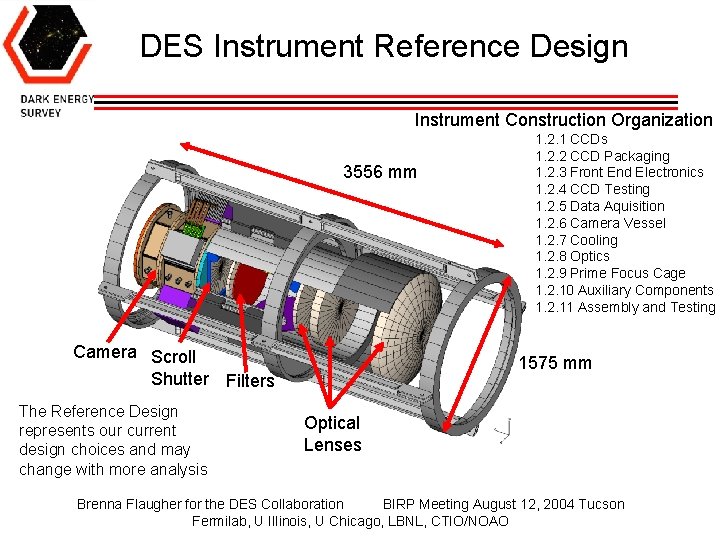
DES Instrument Reference Design Instrument Construction Organization 3556 mm Camera Scroll Shutter Filters The Reference Design represents our current design choices and may change with more analysis 1. 2. 1 CCDs 1. 2. 2 CCD Packaging 1. 2. 3 Front End Electronics 1. 2. 4 CCD Testing 1. 2. 5 Data Aquisition 1. 2. 6 Camera Vessel 1. 2. 7 Cooling 1. 2. 8 Optics 1. 2. 9 Prime Focus Cage 1. 2. 10 Auxiliary Components 1. 2. 11 Assembly and Testing 1575 mm Optical Lenses Brenna Flaugher for the DES Collaboration BIRP Meeting August 12, 2004 Tucson Fermilab, U Illinois, U Chicago, LBNL, CTIO/NOAO
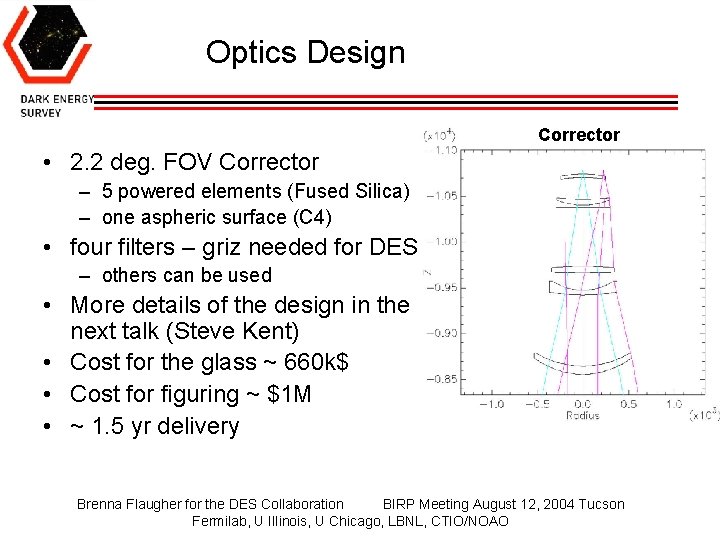
Optics Design Corrector • 2. 2 deg. FOV Corrector – 5 powered elements (Fused Silica) – one aspheric surface (C 4) • four filters – griz needed for DES – others can be used • More details of the design in the next talk (Steve Kent) • Cost for the glass ~ 660 k$ • Cost for figuring ~ $1 M • ~ 1. 5 yr delivery Brenna Flaugher for the DES Collaboration BIRP Meeting August 12, 2004 Tucson Fermilab, U Illinois, U Chicago, LBNL, CTIO/NOAO
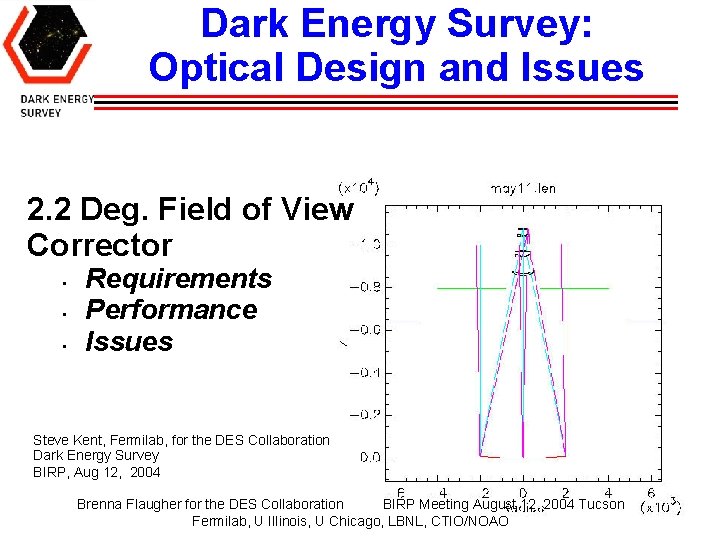
Dark Energy Survey: Optical Design and Issues 2. 2 Deg. Field of View Corrector • • • Requirements Performance Issues Steve Kent, Fermilab, for the DES Collaboration Dark Energy Survey BIRP, Aug 12, 2004 Brenna Flaugher for the DES Collaboration BIRP Meeting August 12, 2004 Tucson Fermilab, U Illinois, U Chicago, LBNL, CTIO/NOAO
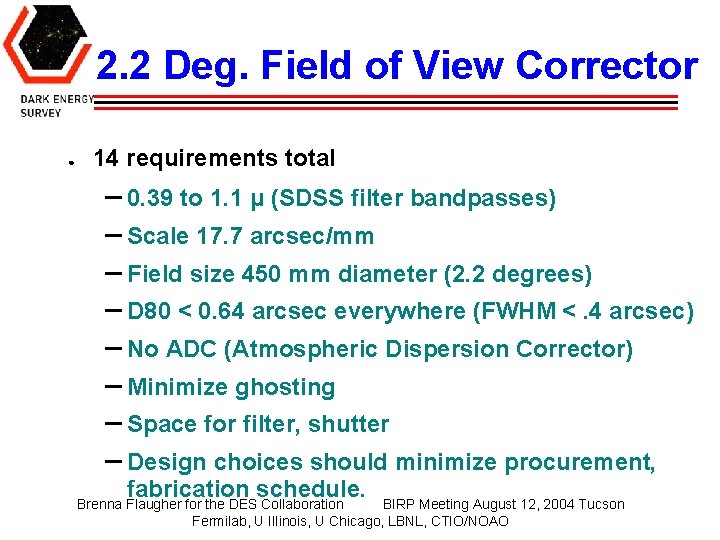
2. 2 Deg. Field of View Corrector ● 14 requirements total – 0. 39 to 1. 1 μ (SDSS filter bandpasses) – Scale 17. 7 arcsec/mm – Field size 450 mm diameter (2. 2 degrees) – D 80 < 0. 64 arcsec everywhere (FWHM <. 4 arcsec) – No ADC (Atmospheric Dispersion Corrector) – Minimize ghosting – Space for filter, shutter – Design choices should minimize procurement, fabrication schedule. Brenna Flaugher for the DES Collaboration BIRP Meeting August 12, 2004 Tucson Fermilab, U Illinois, U Chicago, LBNL, CTIO/NOAO
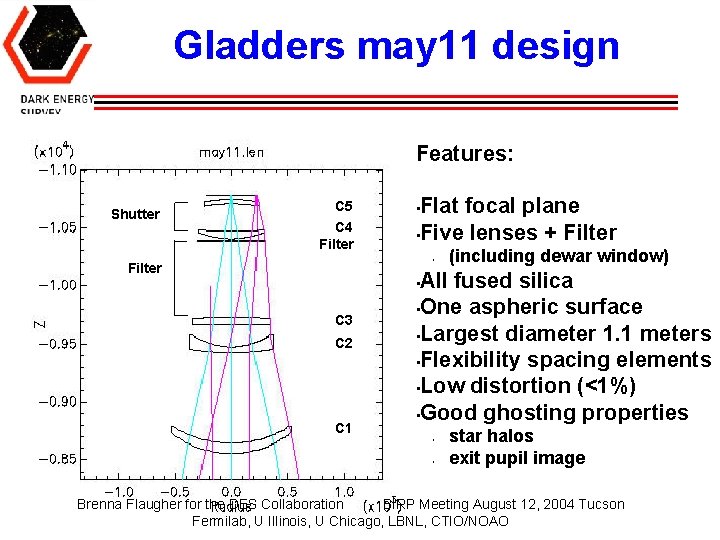
Gladders may 11 design Features: Shutter C 5 C 4 Filter Flat focal plane • Five lenses + Filter • • Filter (including dewar window) All fused silica • One aspheric surface • Largest diameter 1. 1 meters • Flexibility spacing elements • Low distortion (<1%) • Good ghosting properties • C 3 C 2 C 1 • • star halos exit pupil image Brenna Flaugher for the DES Collaboration BIRP Meeting August 12, 2004 Tucson Fermilab, U Illinois, U Chicago, LBNL, CTIO/NOAO
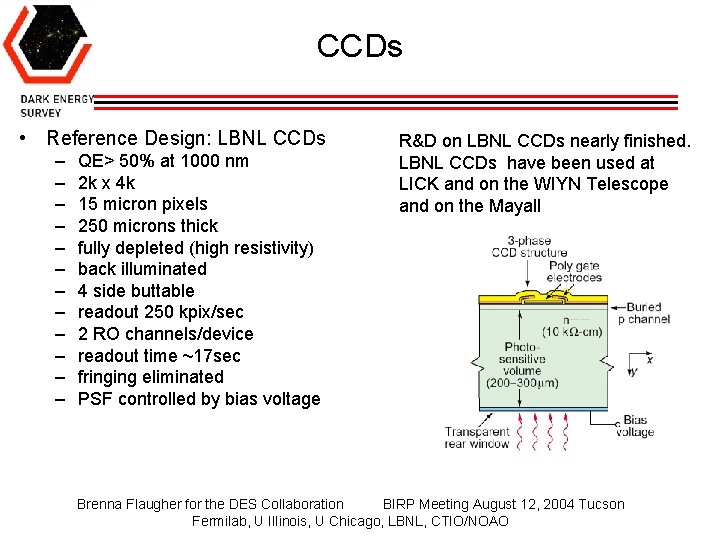
CCDs • Reference Design: LBNL CCDs – – – QE> 50% at 1000 nm 2 k x 4 k 15 micron pixels 250 microns thick fully depleted (high resistivity) back illuminated 4 side buttable readout 250 kpix/sec 2 RO channels/device readout time ~17 sec fringing eliminated PSF controlled by bias voltage R&D on LBNL CCDs nearly finished. LBNL CCDs have been used at LICK and on the WIYN Telescope and on the Mayall Brenna Flaugher for the DES Collaboration BIRP Meeting August 12, 2004 Tucson Fermilab, U Illinois, U Chicago, LBNL, CTIO/NOAO
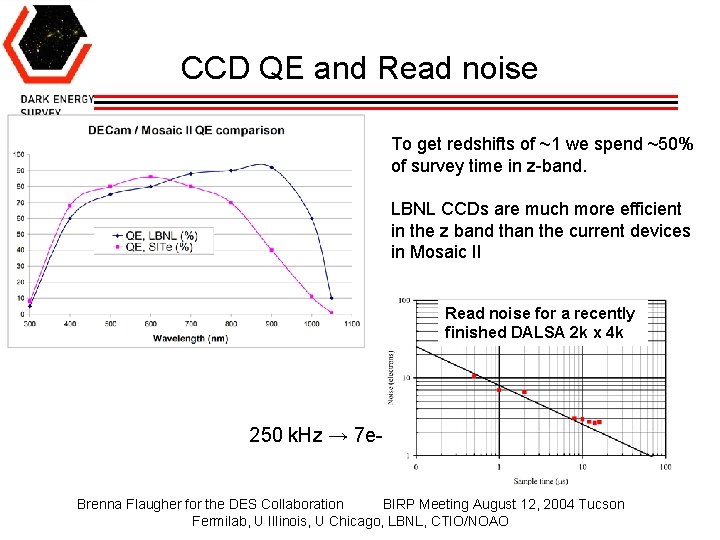
CCD QE and Read noise To get redshifts of ~1 we spend ~50% of survey time in z-band. LBNL CCDs are much more efficient in the z band than the current devices in Mosaic II Read noise for a recently finished DALSA 2 k x 4 k 250 k. Hz → 7 e- Brenna Flaugher for the DES Collaboration BIRP Meeting August 12, 2004 Tucson Fermilab, U Illinois, U Chicago, LBNL, CTIO/NOAO
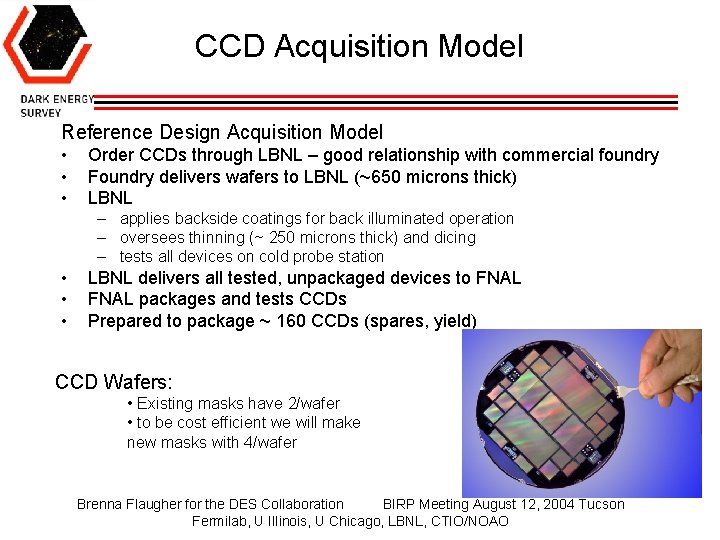
CCD Acquisition Model Reference Design Acquisition Model • • • Order CCDs through LBNL – good relationship with commercial foundry Foundry delivers wafers to LBNL (~650 microns thick) LBNL – applies backside coatings for back illuminated operation – oversees thinning (~ 250 microns thick) and dicing – tests all devices on cold probe station • • • LBNL delivers all tested, unpackaged devices to FNAL packages and tests CCDs Prepared to package ~ 160 CCDs (spares, yield) CCD Wafers: • Existing masks have 2/wafer • to be cost efficient we will make new masks with 4/wafer Brenna Flaugher for the DES Collaboration BIRP Meeting August 12, 2004 Tucson Fermilab, U Illinois, U Chicago, LBNL, CTIO/NOAO
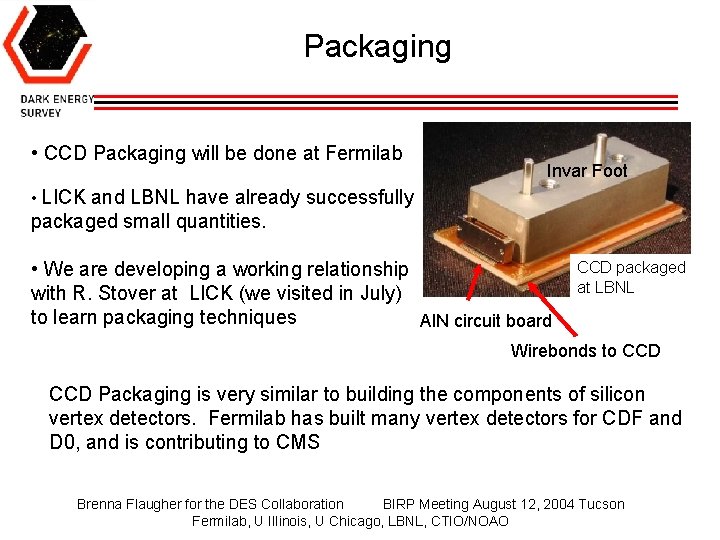
Packaging • CCD Packaging will be done at Fermilab Invar Foot • LICK and LBNL have already successfully packaged small quantities. • We are developing a working relationship with R. Stover at LICK (we visited in July) to learn packaging techniques Al. N circuit board CCD packaged at LBNL Wirebonds to CCD Packaging is very similar to building the components of silicon vertex detectors. Fermilab has built many vertex detectors for CDF and D 0, and is contributing to CMS Brenna Flaugher for the DES Collaboration BIRP Meeting August 12, 2004 Tucson Fermilab, U Illinois, U Chicago, LBNL, CTIO/NOAO
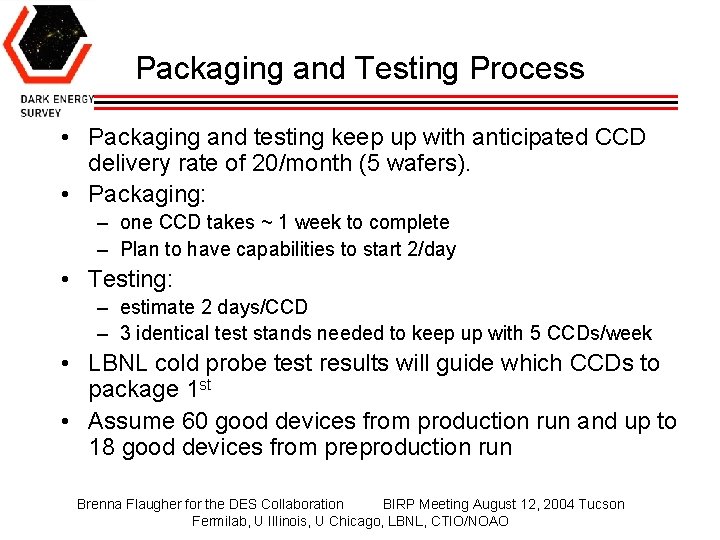
Packaging and Testing Process • Packaging and testing keep up with anticipated CCD delivery rate of 20/month (5 wafers). • Packaging: – one CCD takes ~ 1 week to complete – Plan to have capabilities to start 2/day • Testing: – estimate 2 days/CCD – 3 identical test stands needed to keep up with 5 CCDs/week • LBNL cold probe test results will guide which CCDs to package 1 st • Assume 60 good devices from production run and up to 18 good devices from preproduction run Brenna Flaugher for the DES Collaboration BIRP Meeting August 12, 2004 Tucson Fermilab, U Illinois, U Chicago, LBNL, CTIO/NOAO
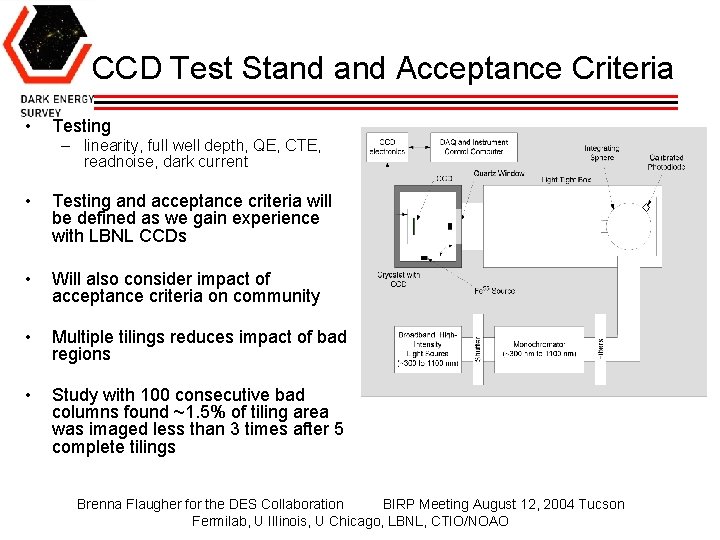
CCD Test Stand Acceptance Criteria • Testing – linearity, full well depth, QE, CTE, readnoise, dark current • Testing and acceptance criteria will be defined as we gain experience with LBNL CCDs • Will also consider impact of acceptance criteria on community • Multiple tilings reduces impact of bad regions • Study with 100 consecutive bad columns found ~1. 5% of tiling area was imaged less than 3 times after 5 complete tilings Brenna Flaugher for the DES Collaboration BIRP Meeting August 12, 2004 Tucson Fermilab, U Illinois, U Chicago, LBNL, CTIO/NOAO
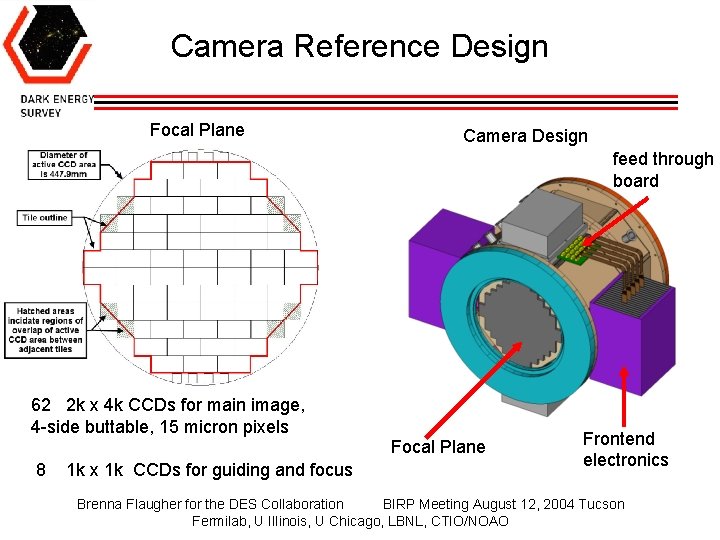
Camera Reference Design Focal Plane Camera Design feed through board 62 2 k x 4 k CCDs for main image, 4 -side buttable, 15 micron pixels Focal Plane 8 1 k x 1 k CCDs for guiding and focus Frontend electronics Brenna Flaugher for the DES Collaboration BIRP Meeting August 12, 2004 Tucson Fermilab, U Illinois, U Chicago, LBNL, CTIO/NOAO
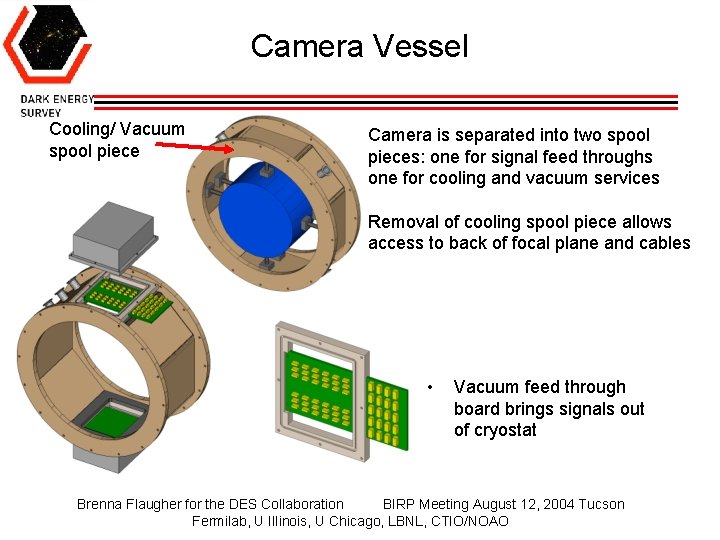
Camera Vessel Cooling/ Vacuum spool piece Camera is separated into two spool pieces: one for signal feed throughs one for cooling and vacuum services Removal of cooling spool piece allows access to back of focal plane and cables • Vacuum feed through board brings signals out of cryostat Brenna Flaugher for the DES Collaboration BIRP Meeting August 12, 2004 Tucson Fermilab, U Illinois, U Chicago, LBNL, CTIO/NOAO
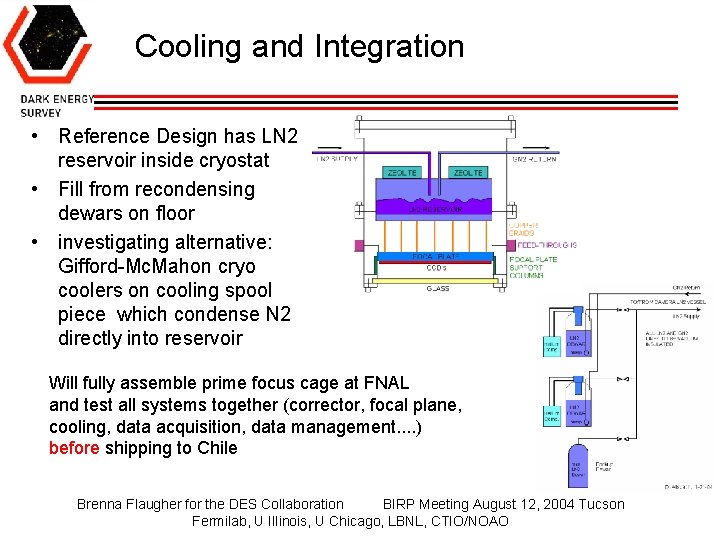
Cooling and Integration • Reference Design has LN 2 reservoir inside cryostat • Fill from recondensing dewars on floor • investigating alternative: Gifford-Mc. Mahon cryo coolers on cooling spool piece which condense N 2 directly into reservoir Will fully assemble prime focus cage at FNAL and test all systems together (corrector, focal plane, cooling, data acquisition, data management. . ) before shipping to Chile Brenna Flaugher for the DES Collaboration BIRP Meeting August 12, 2004 Tucson Fermilab, U Illinois, U Chicago, LBNL, CTIO/NOAO
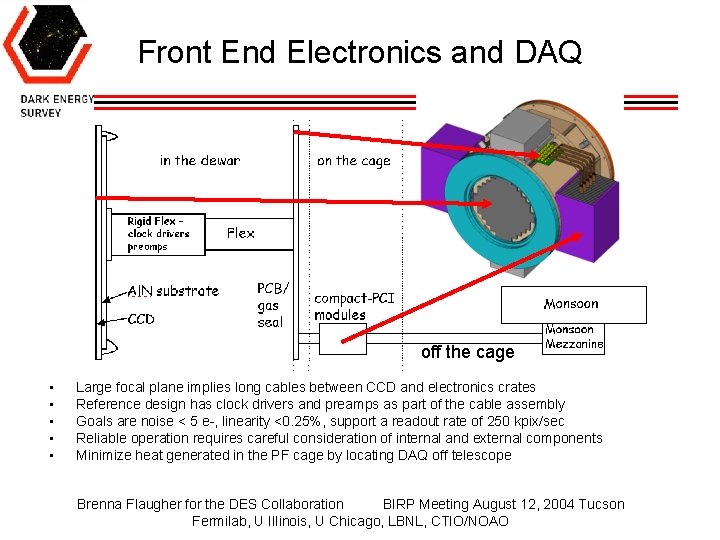
Front End Electronics and DAQ off the cage • • • Large focal plane implies long cables between CCD and electronics crates Reference design has clock drivers and preamps as part of the cable assembly Goals are noise < 5 e-, linearity <0. 25%, support a readout rate of 250 kpix/sec Reliable operation requires careful consideration of internal and external components Minimize heat generated in the PF cage by locating DAQ off telescope Brenna Flaugher for the DES Collaboration BIRP Meeting August 12, 2004 Tucson Fermilab, U Illinois, U Chicago, LBNL, CTIO/NOAO
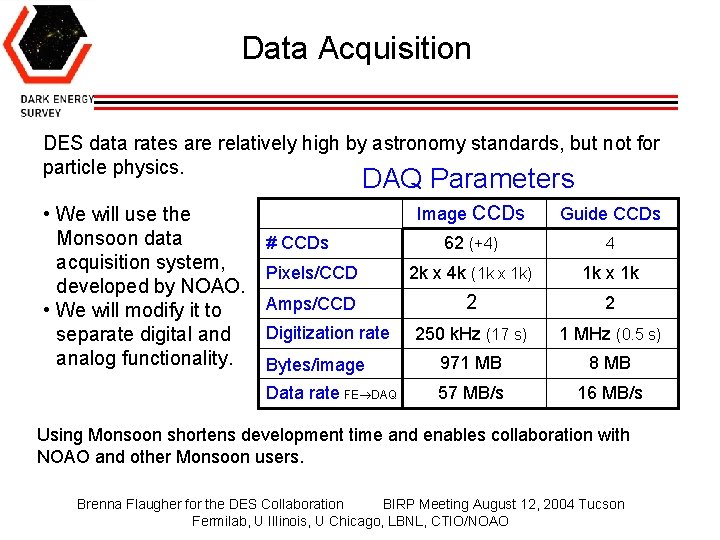
Data Acquisition DES data rates are relatively high by astronomy standards, but not for particle physics. DAQ Parameters • We will use the Monsoon data acquisition system, developed by NOAO. • We will modify it to separate digital and analog functionality. Image CCDs Guide CCDs 62 (+4) 4 Pixels/CCD 2 k x 4 k (1 k x 1 k) 1 k x 1 k Amps/CCD 2 2 250 k. Hz (17 s) 1 MHz (0. 5 s) Bytes/image 971 MB 8 MB Data rate FE DAQ 57 MB/s 16 MB/s # CCDs Digitization rate Using Monsoon shortens development time and enables collaboration with NOAO and other Monsoon users. Brenna Flaugher for the DES Collaboration BIRP Meeting August 12, 2004 Tucson Fermilab, U Illinois, U Chicago, LBNL, CTIO/NOAO
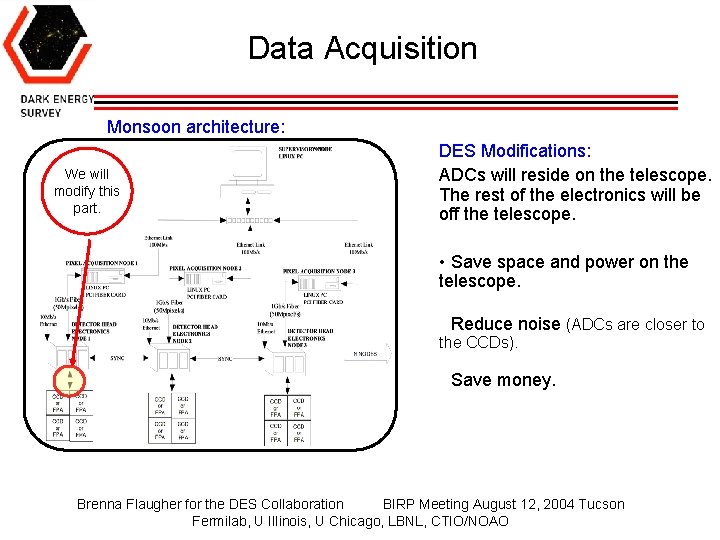
Data Acquisition Monsoon architecture: We will modify this part. DES Modifications: ADCs will reside on the telescope. The rest of the electronics will be off the telescope. • Save space and power on the telescope. • Reduce noise (ADCs are closer to the CCDs). • Save money. Brenna Flaugher for the DES Collaboration BIRP Meeting August 12, 2004 Tucson Fermilab, U Illinois, U Chicago, LBNL, CTIO/NOAO
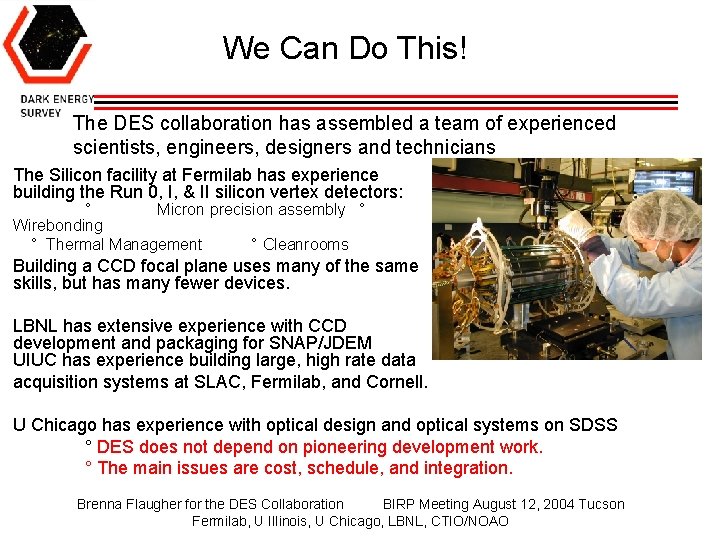
We Can Do This! The DES collaboration has assembled a team of experienced scientists, engineers, designers and technicians The Silicon facility at Fermilab has experience building the Run 0, I, & II silicon vertex detectors: ° Micron precision assembly ° Wirebonding ° Thermal Management ° Cleanrooms Building a CCD focal plane uses many of the same skills, but has many fewer devices. LBNL has extensive experience with CCD development and packaging for SNAP/JDEM UIUC has experience building large, high rate data acquisition systems at SLAC, Fermilab, and Cornell. U Chicago has experience with optical design and optical systems on SDSS ° DES does not depend on pioneering development work. ° The main issues are cost, schedule, and integration. Brenna Flaugher for the DES Collaboration BIRP Meeting August 12, 2004 Tucson Fermilab, U Illinois, U Chicago, LBNL, CTIO/NOAO
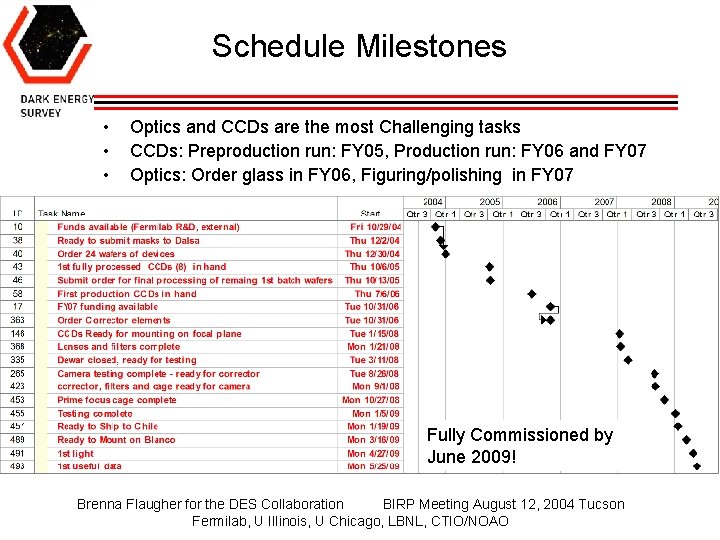
Schedule Milestones • • • Optics and CCDs are the most Challenging tasks CCDs: Preproduction run: FY 05, Production run: FY 06 and FY 07 Optics: Order glass in FY 06, Figuring/polishing in FY 07 Fully Commissioned by June 2009! Brenna Flaugher for the DES Collaboration BIRP Meeting August 12, 2004 Tucson Fermilab, U Illinois, U Chicago, LBNL, CTIO/NOAO
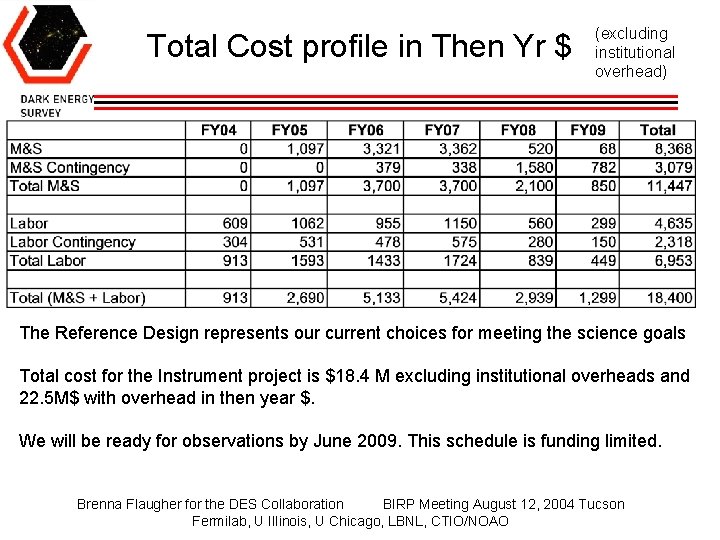
Total Cost profile in Then Yr $ (excluding institutional overhead) The Reference Design represents our current choices for meeting the science goals Total cost for the Instrument project is $18. 4 M excluding institutional overheads and 22. 5 M$ with overhead in then year $. We will be ready for observations by June 2009. This schedule is funding limited. Brenna Flaugher for the DES Collaboration BIRP Meeting August 12, 2004 Tucson Fermilab, U Illinois, U Chicago, LBNL, CTIO/NOAO
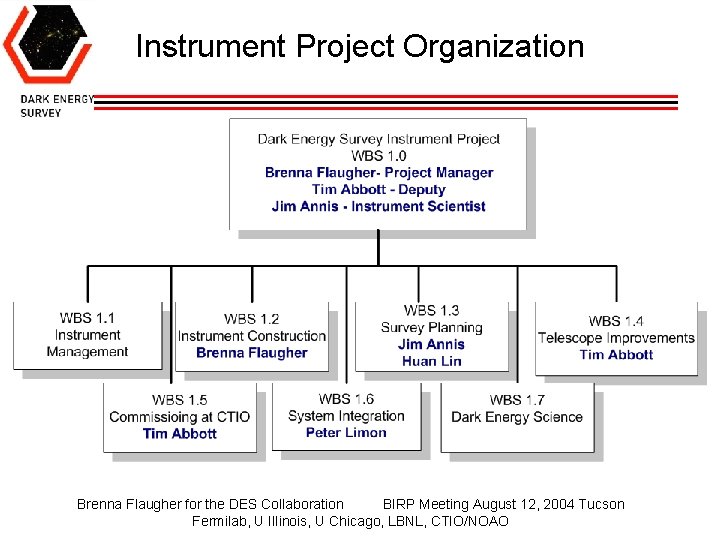
Instrument Project Organization Brenna Flaugher for the DES Collaboration BIRP Meeting August 12, 2004 Tucson Fermilab, U Illinois, U Chicago, LBNL, CTIO/NOAO
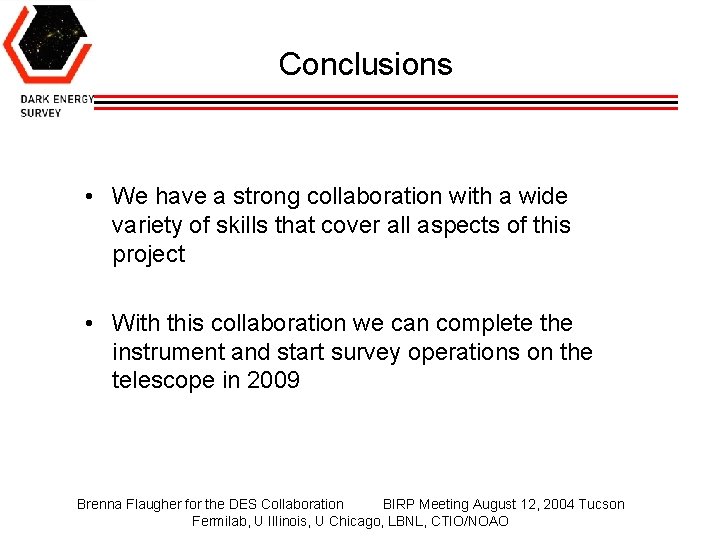
Conclusions • We have a strong collaboration with a wide variety of skills that cover all aspects of this project • With this collaboration we can complete the instrument and start survey operations on the telescope in 2009 Brenna Flaugher for the DES Collaboration BIRP Meeting August 12, 2004 Tucson Fermilab, U Illinois, U Chicago, LBNL, CTIO/NOAO
Dark matter and dark energy presentation
Dr. francisco javier kuri con
Francisco javier salazar silva
Colegio san francisco javier
Colegio san francisco javier
Francisco javier robles de mendoza
Francisco javier tapia greave
Escuela secundaria tecnica 60 francisco javier santamaria
Des des des
In a dark dark town
Acls primary survey
Energy energy transfer and general energy analysis
Energy energy transfer and general energy analysis
Cube of stabilized dark energy
Dma dark energy
La diffusion des idées des lumières
Il existe des personnes qui sont des lumières pour tous
Valeur des temps verbaux
Plan marshall affiche
Diversification des espaces et des acteurs de la production
Volume d un corps
Volume des liquides et des solides
Cartographie des risques gestion des stocks
Je t'offrirai des fleurs et des nappes en couleurs
Robin des bois des alpes
Des in networking
Budget des ventes méthode des moindres carrés
Javier arvalo
Javier navaridas
Javier remiro
Dr. javier collado
Javier arvalo
Dr. javier e. calvo
Javier salek
Cual es el dominio
Javier canillas
"joshua wu kai-ming"
5 whys washington monument
Javier royo psiquiatra consulta
Impenetrabilidad
Javier navaridas
Javier eli dominguez hernandez
Nelson javier duenas gil
Dr javier campuzano
Javier cervera dentiste
Javier mateo-sagasta
Valoración abc
Características de una entrevista
Juan javier sanchez zavala
Javier arregui
Em tiffany
La javier
Mario javier ojeda hernandez
Carnicería javier
Javier pilar
Javier royo moya
Javier ocasio-perez
Dr javier barrera
Tipo de letras