Chapter 2 Reciprocal Lattice Issues that are addressed
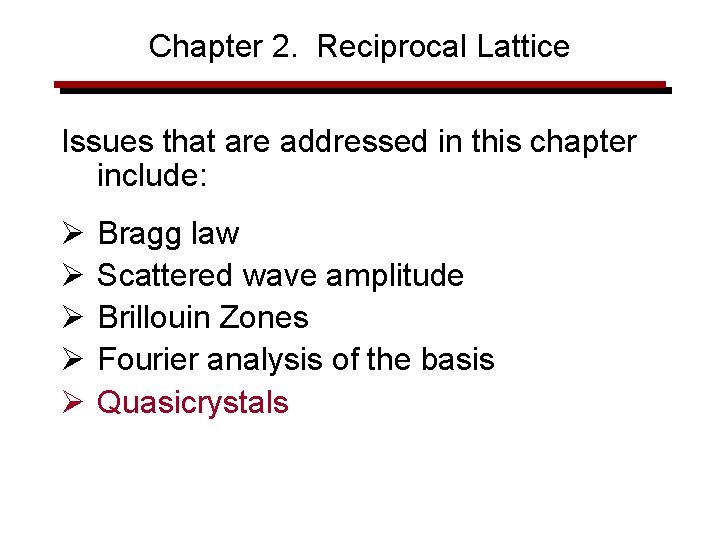
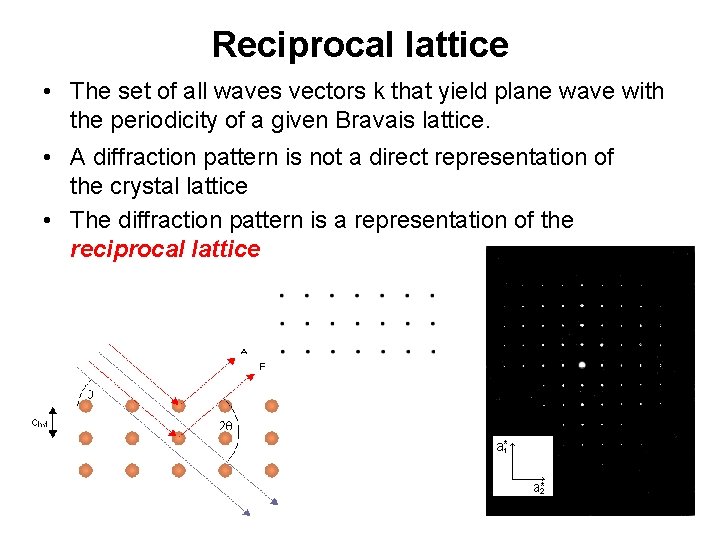
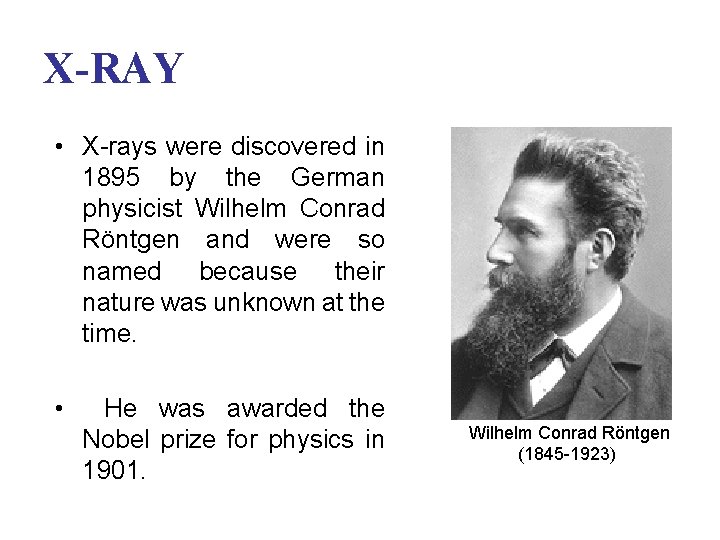
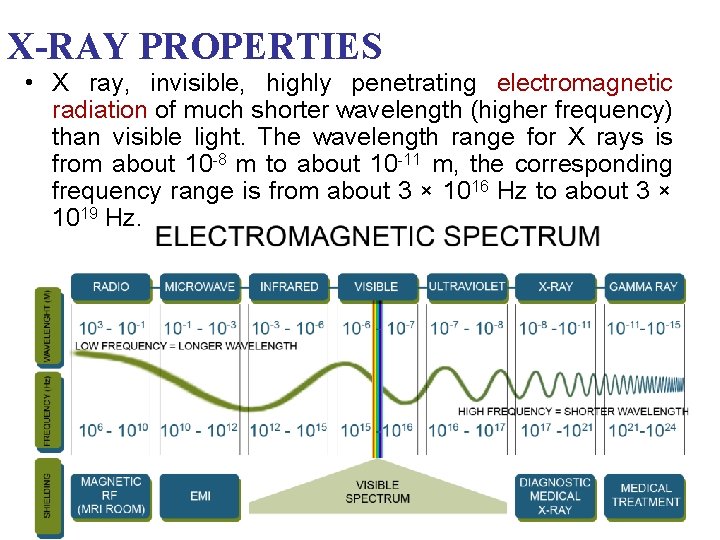
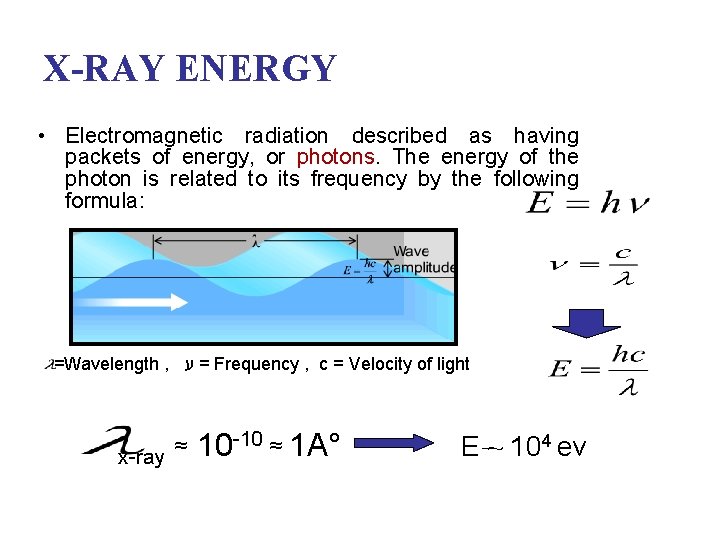
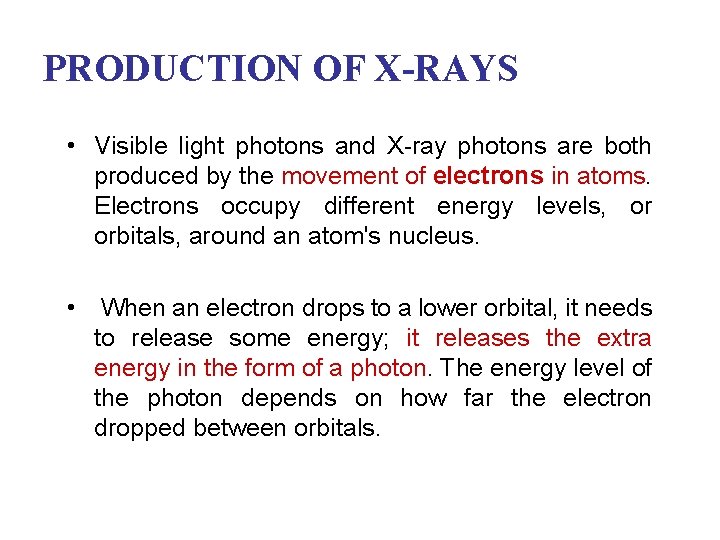
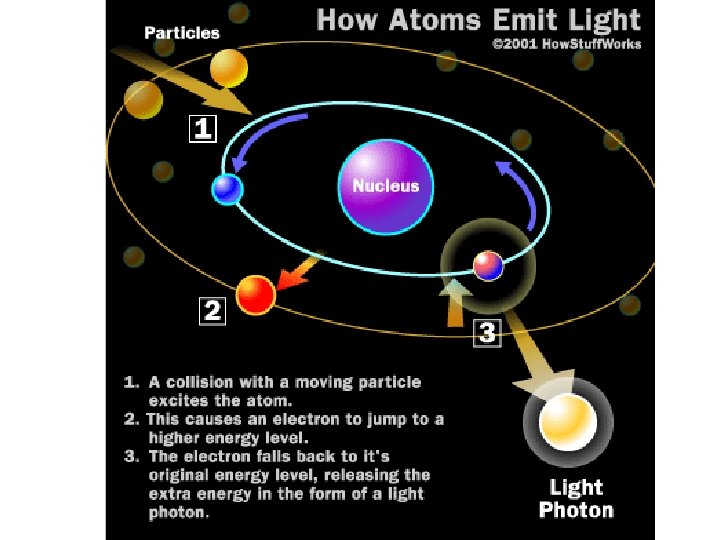
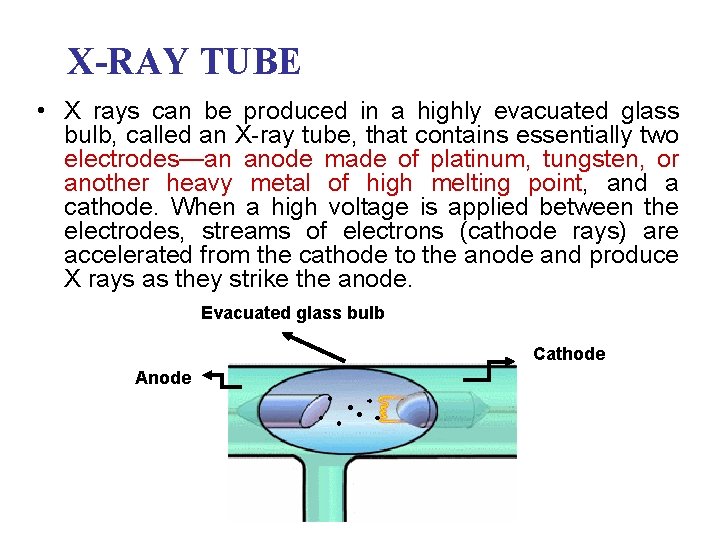
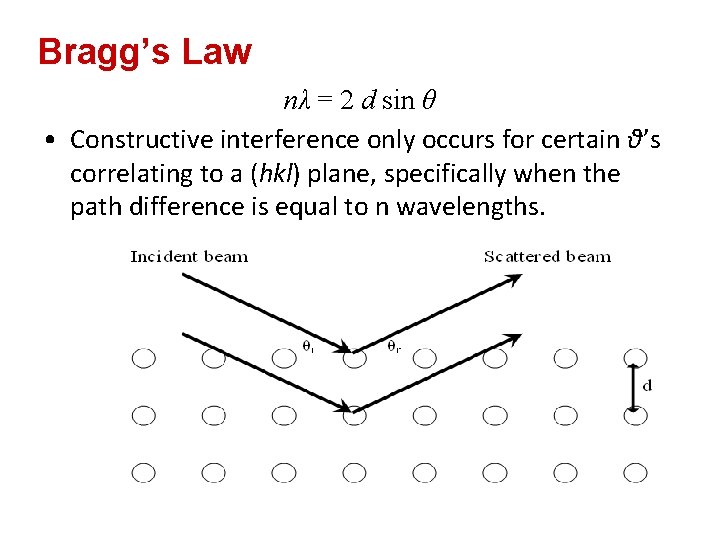
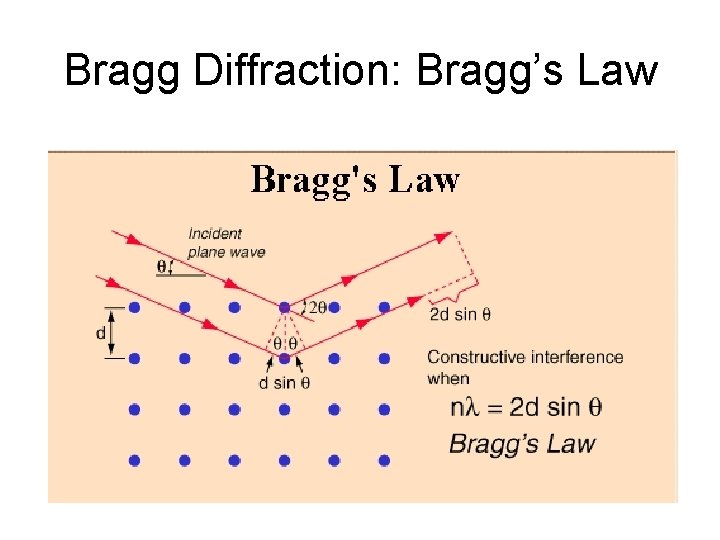
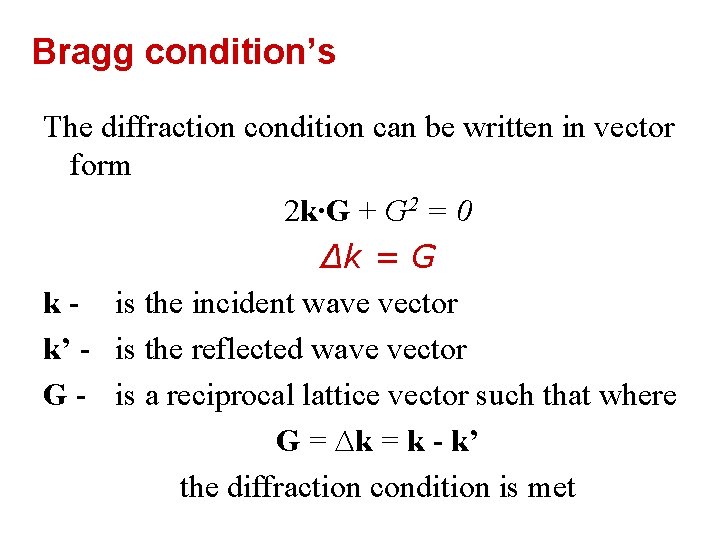
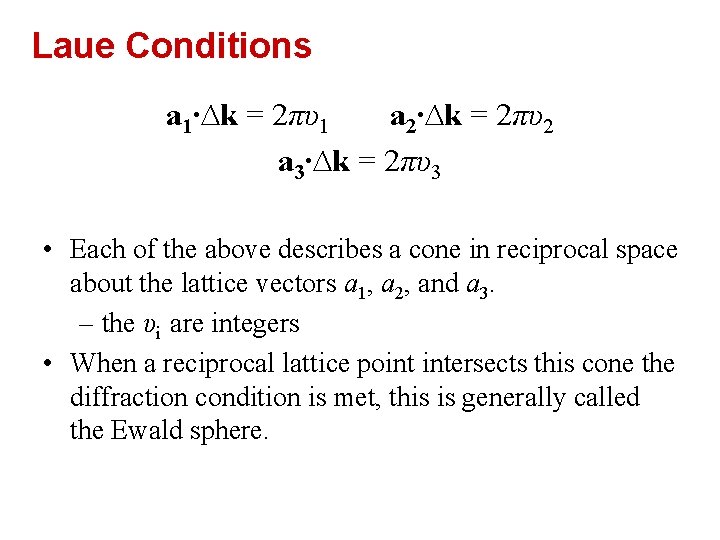
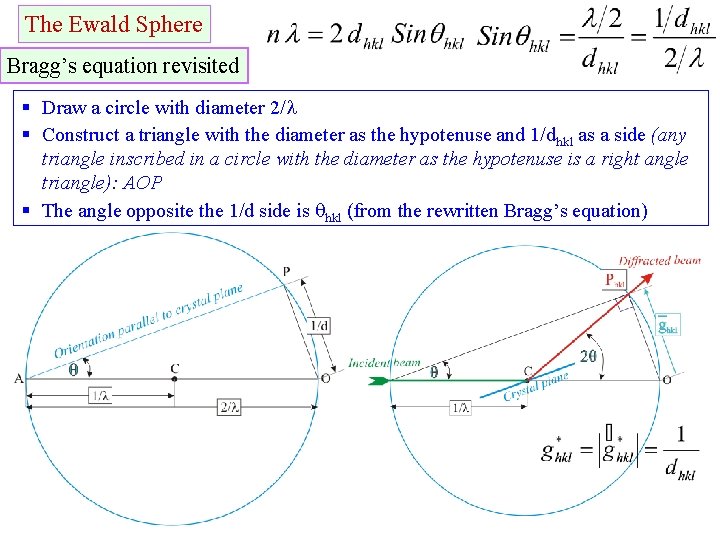
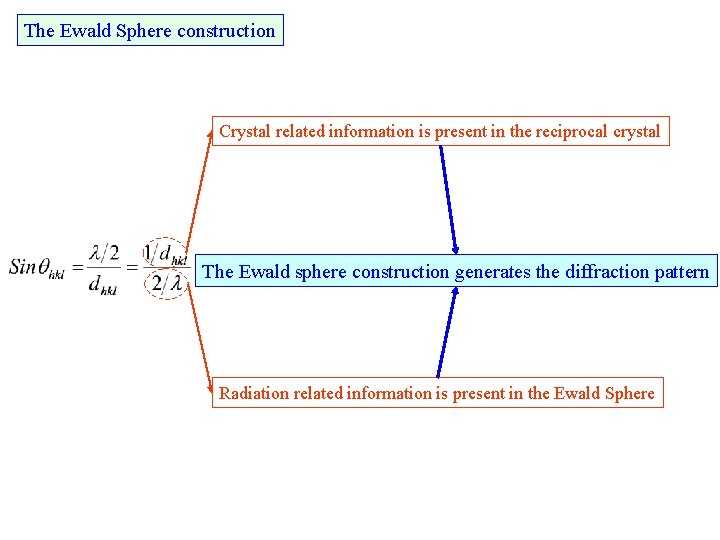
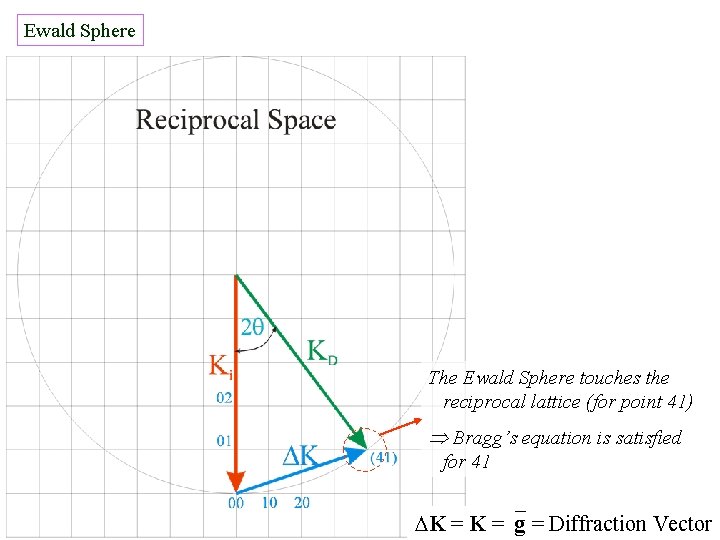
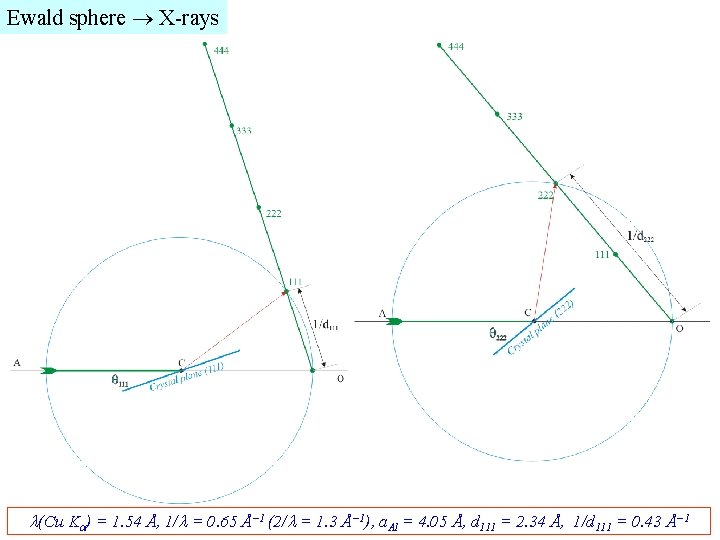
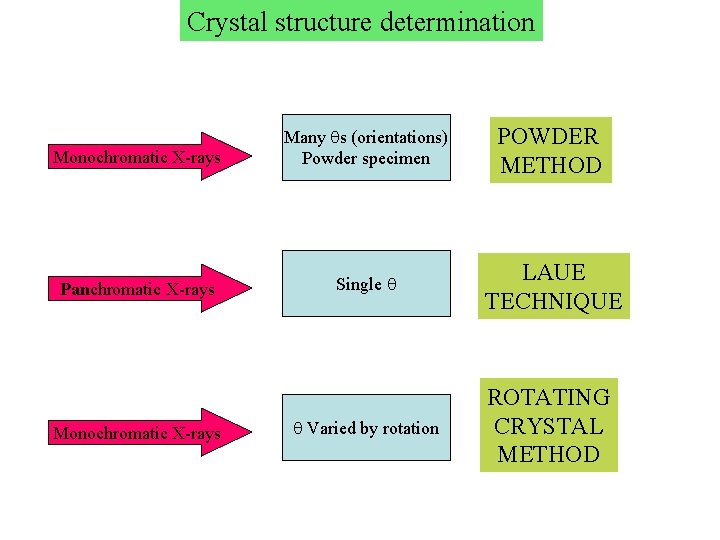
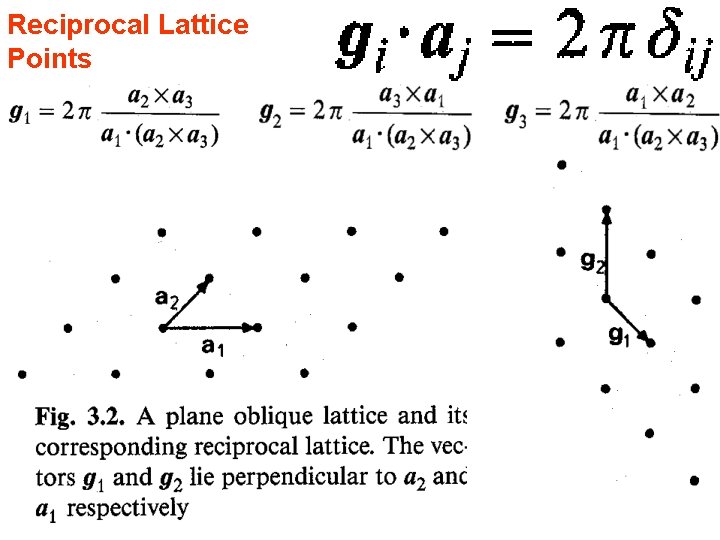
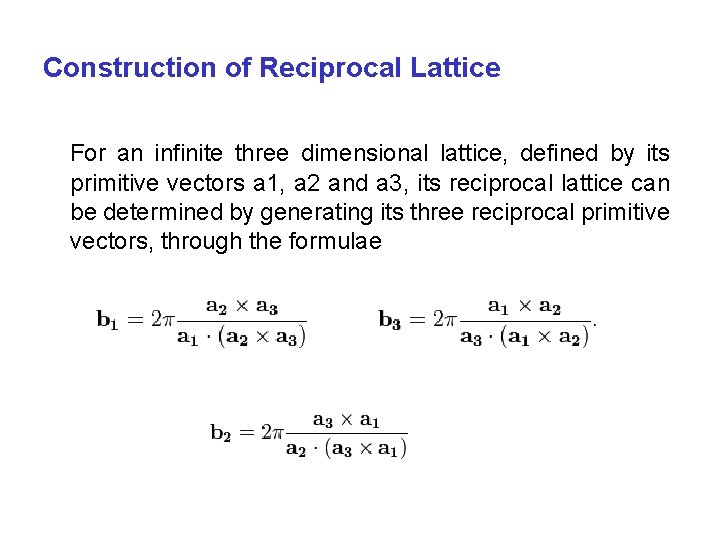
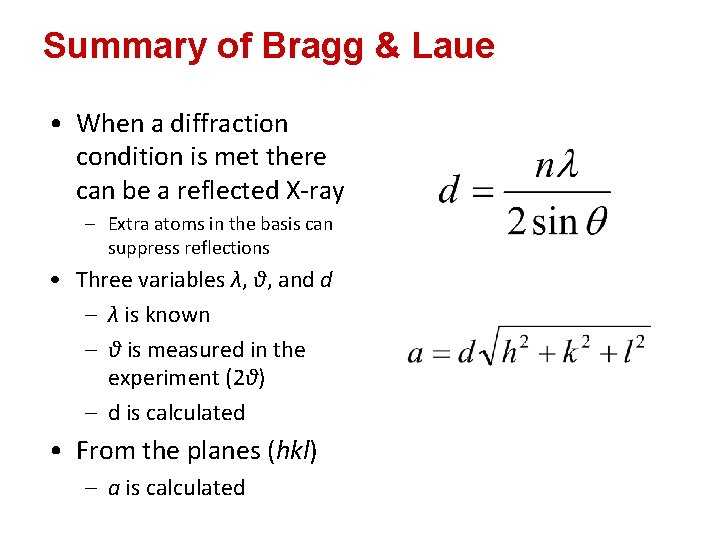
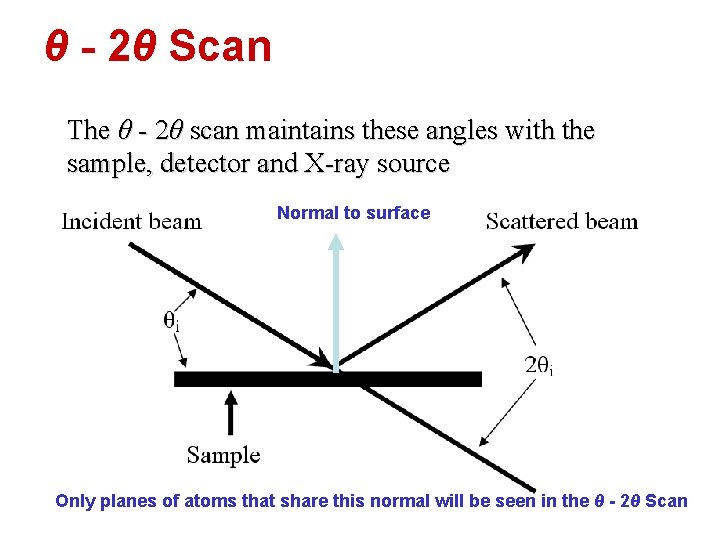
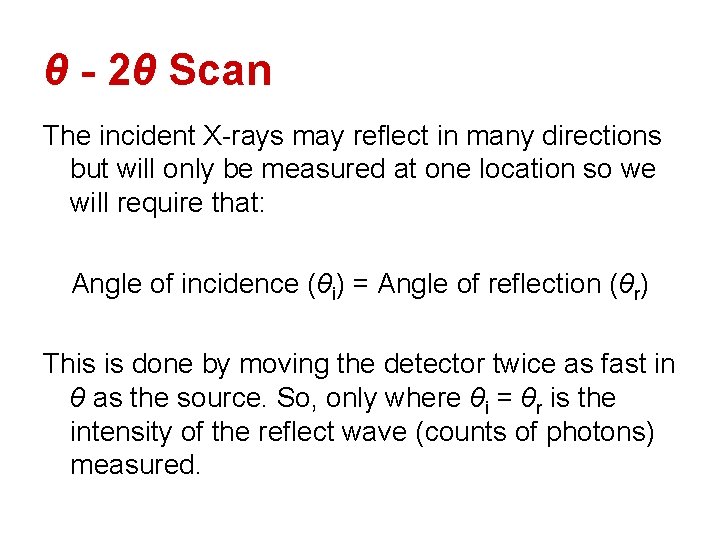
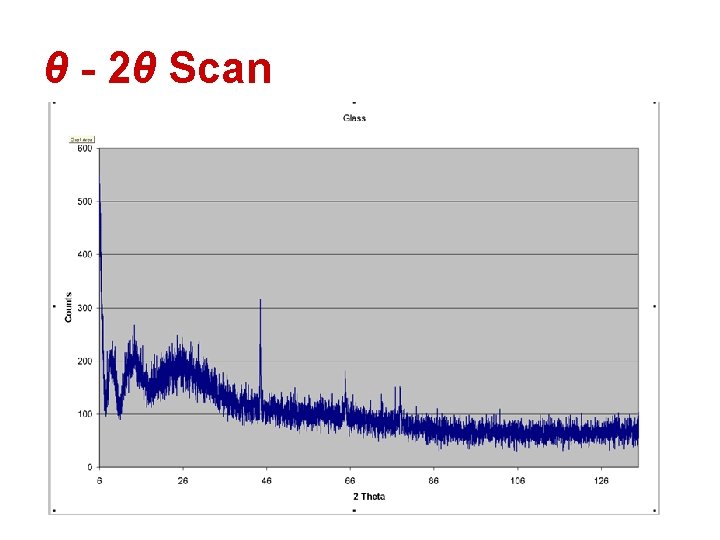
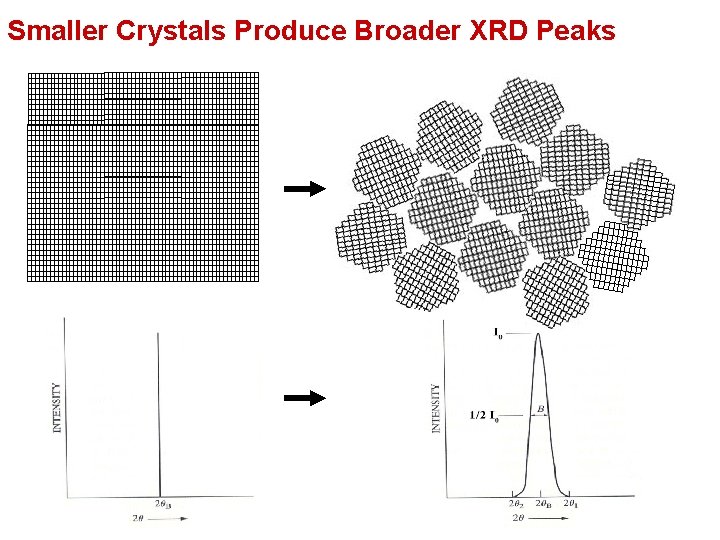
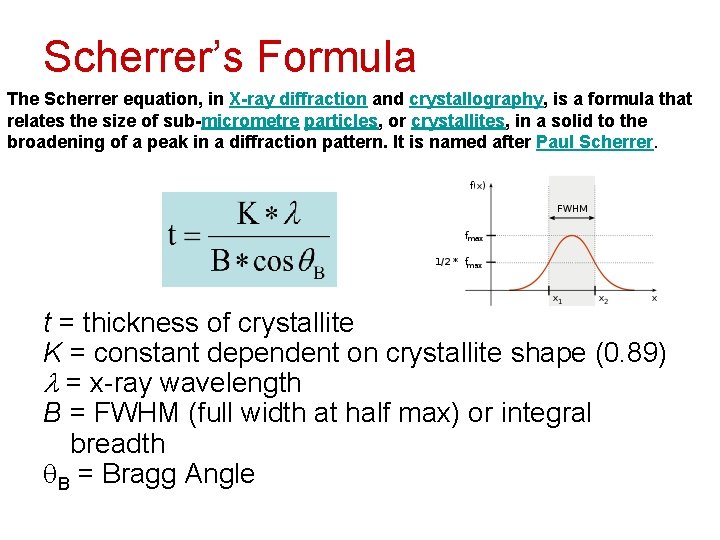
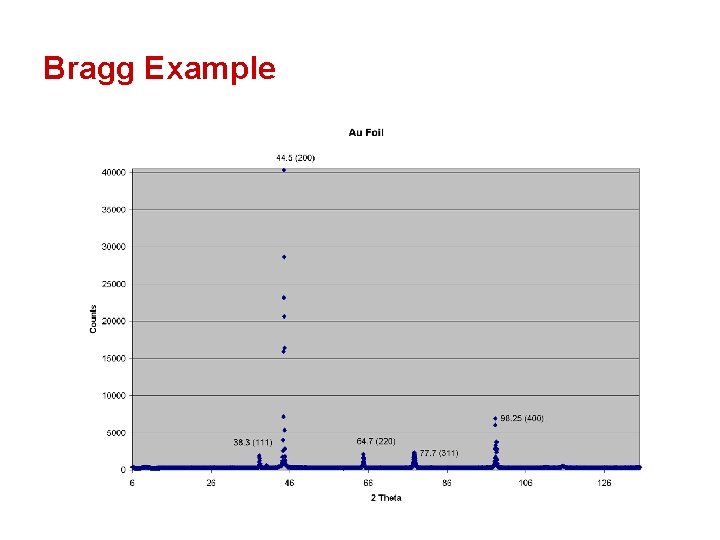
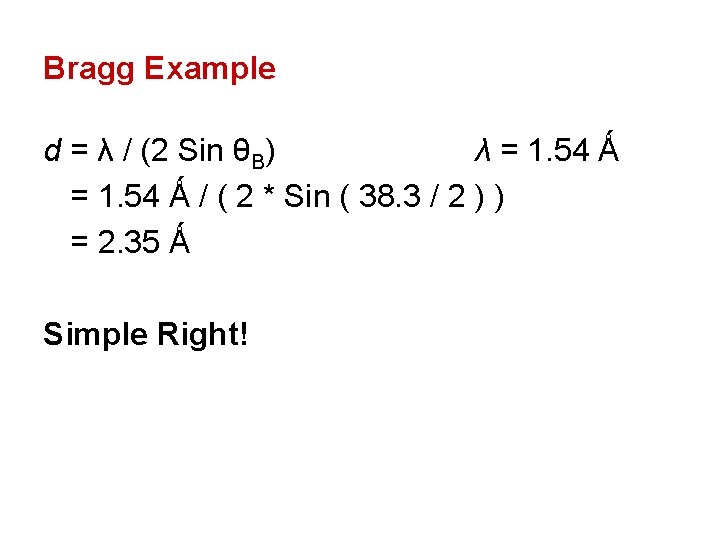
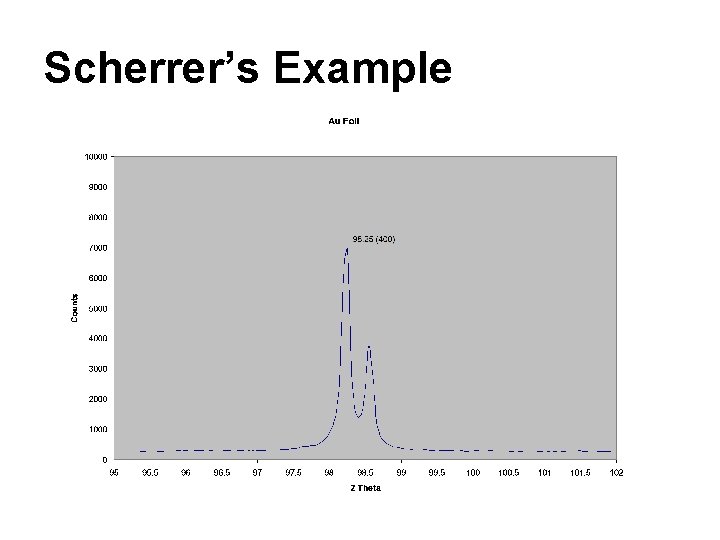
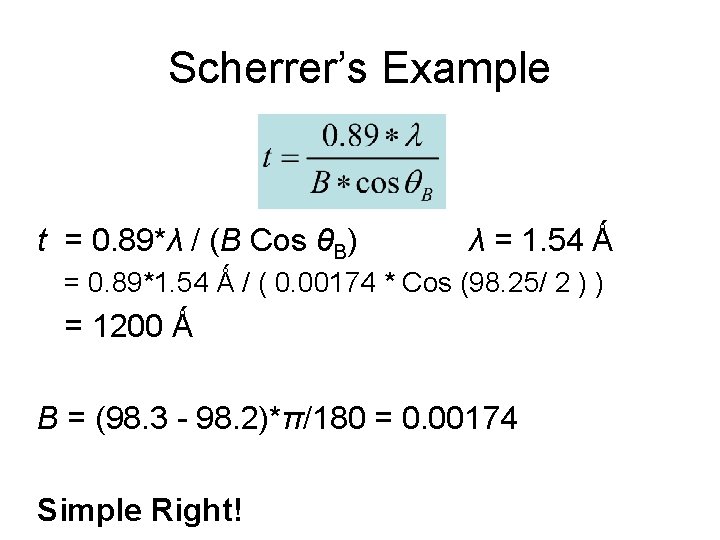
- Slides: 29
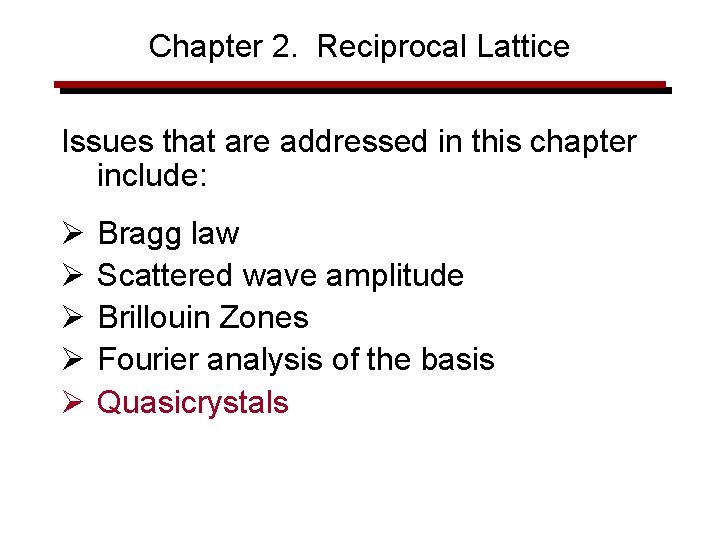
Chapter 2. Reciprocal Lattice Issues that are addressed in this chapter include: Ø Ø Ø Bragg law Scattered wave amplitude Brillouin Zones Fourier analysis of the basis Quasicrystals
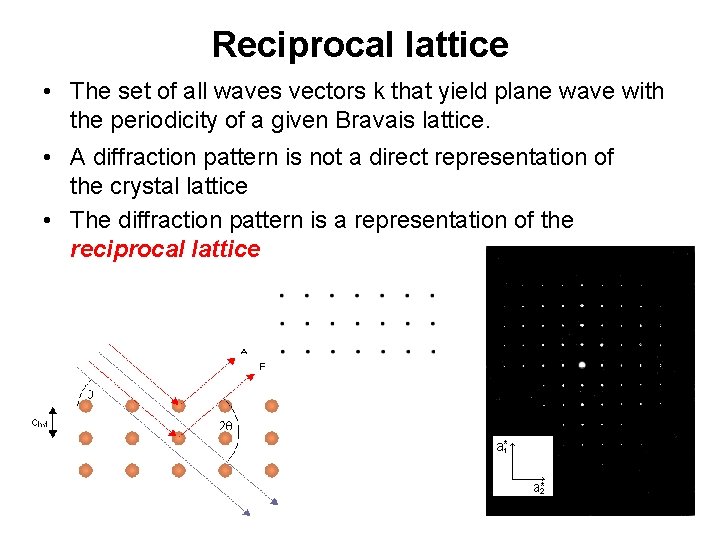
Reciprocal lattice • The set of all waves vectors k that yield plane wave with the periodicity of a given Bravais lattice. • A diffraction pattern is not a direct representation of the crystal lattice • The diffraction pattern is a representation of the reciprocal lattice
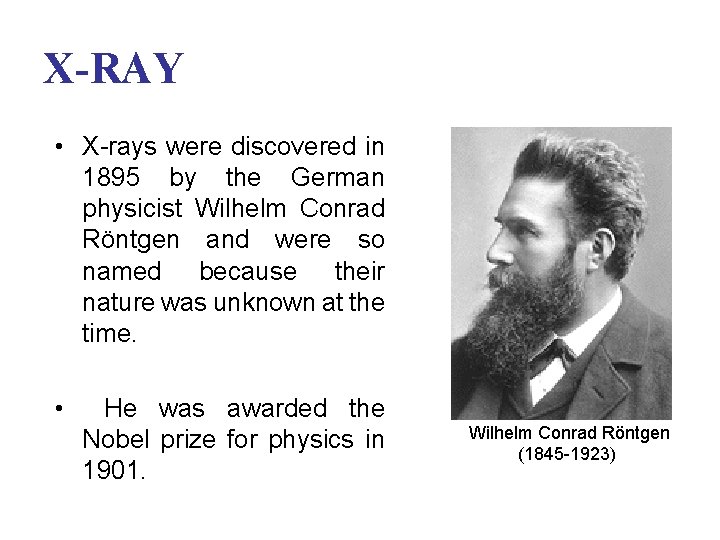
X-RAY • X-rays were discovered in 1895 by the German physicist Wilhelm Conrad Röntgen and were so named because their nature was unknown at the time. • He was awarded the Nobel prize for physics in 1901. Wilhelm Conrad Röntgen (1845 -1923)
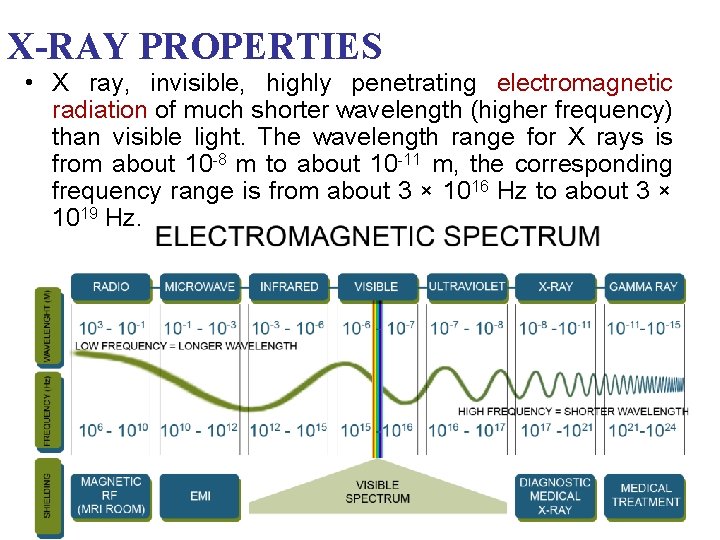
X-RAY PROPERTIES • X ray, invisible, highly penetrating electromagnetic radiation of much shorter wavelength (higher frequency) than visible light. The wavelength range for X rays is from about 10 -8 m to about 10 -11 m, the corresponding frequency range is from about 3 × 1016 Hz to about 3 × 1019 Hz.
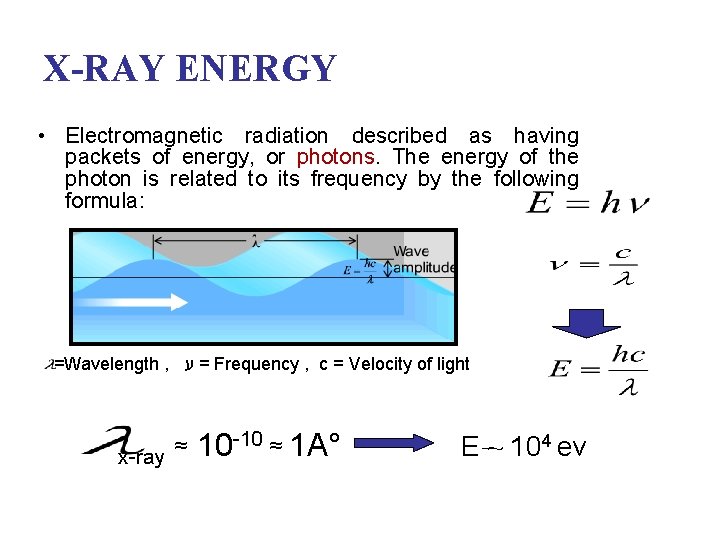
X-RAY ENERGY • Electromagnetic radiation described as having packets of energy, or photons. The energy of the photon is related to its frequency by the following formula: =Wavelength , = ע Frequency , c = Velocity of light x-ray ≈ 10 -10 ≈ 1 A° E ~ 104 ev
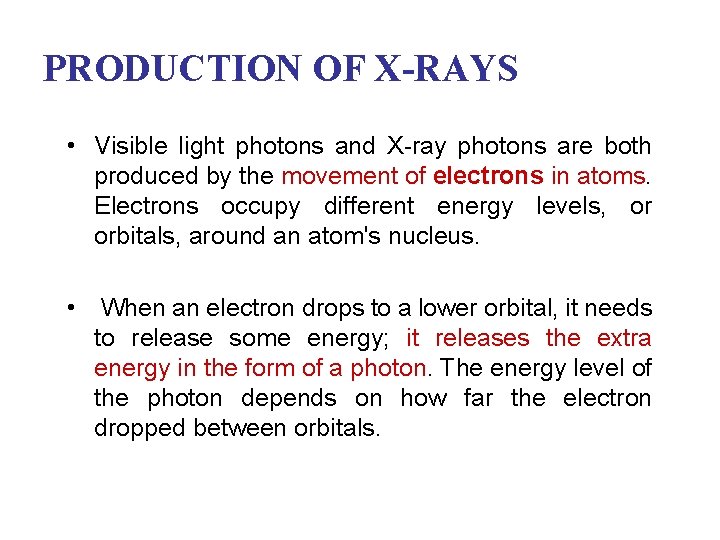
PRODUCTION OF X-RAYS • Visible light photons and X-ray photons are both produced by the movement of electrons in atoms. Electrons occupy different energy levels, or orbitals, around an atom's nucleus. • When an electron drops to a lower orbital, it needs to release some energy; it releases the extra energy in the form of a photon. The energy level of the photon depends on how far the electron dropped between orbitals.
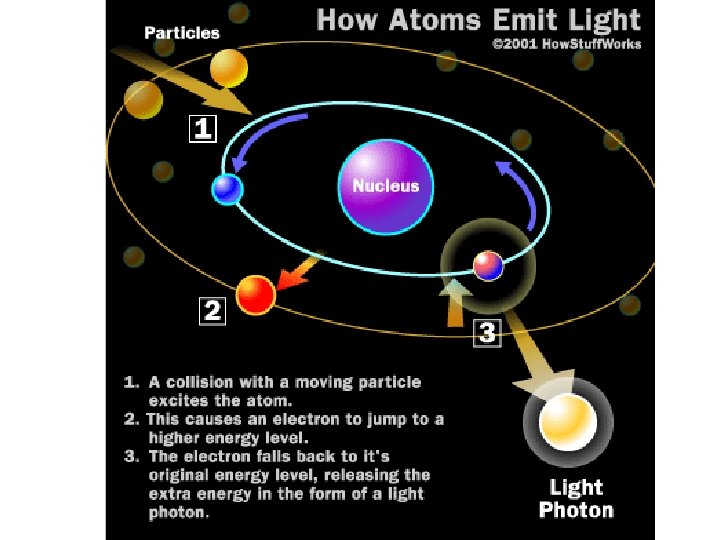
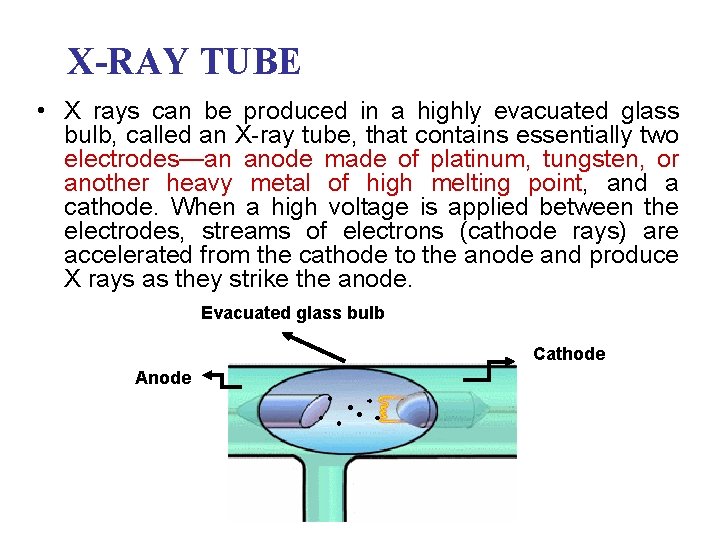
X-RAY TUBE • X rays can be produced in a highly evacuated glass bulb, called an X-ray tube, that contains essentially two electrodes—an anode made of platinum, tungsten, or another heavy metal of high melting point, and a cathode. When a high voltage is applied between the electrodes, streams of electrons (cathode rays) are accelerated from the cathode to the anode and produce X rays as they strike the anode. Evacuated glass bulb Cathode Anode
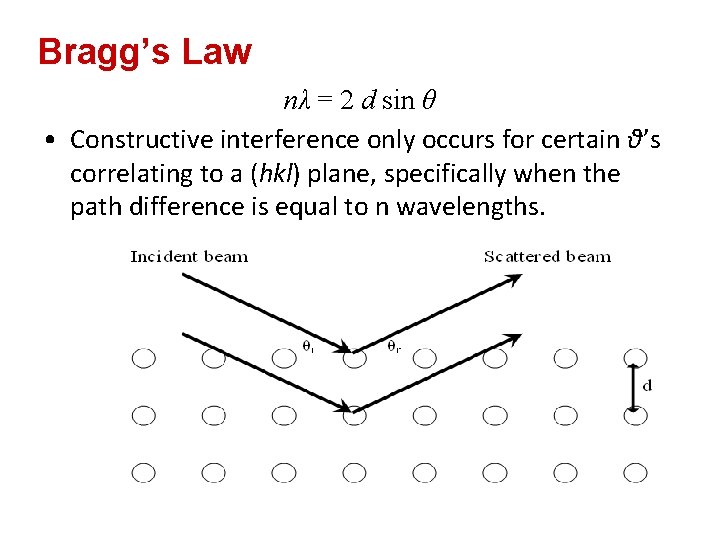
Bragg’s Law nλ = 2 d sin θ • Constructive interference only occurs for certain θ’s correlating to a (hkl) plane, specifically when the path difference is equal to n wavelengths.
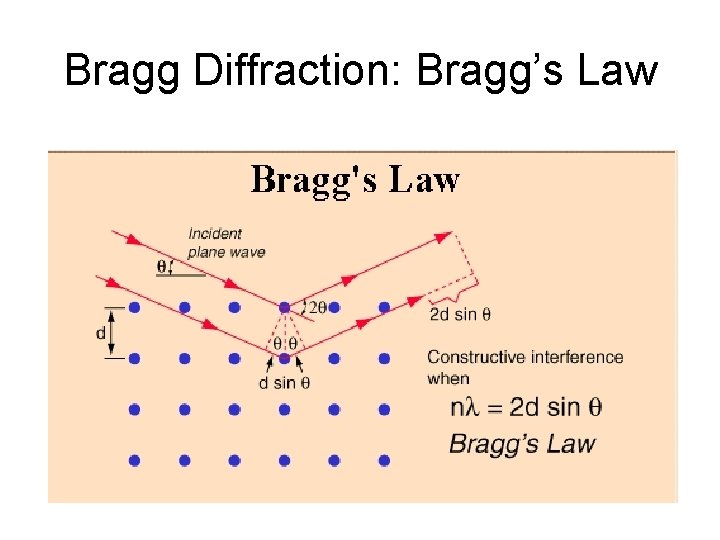
Bragg Diffraction: Bragg’s Law
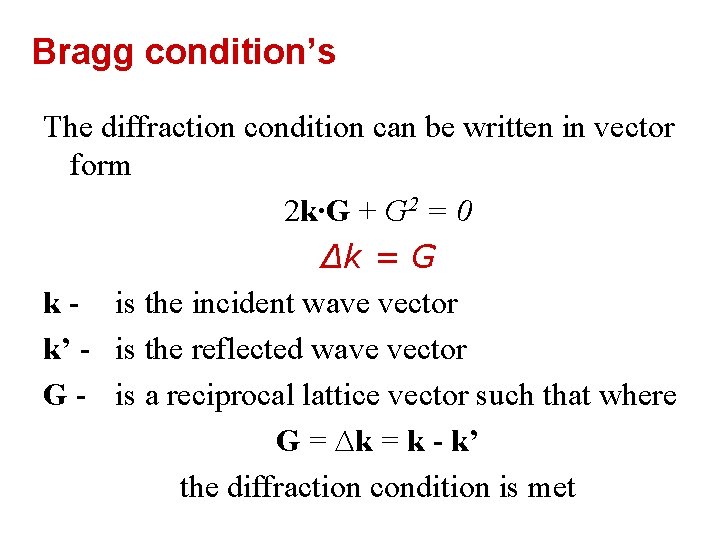
Bragg condition’s The diffraction condition can be written in vector form 2 k∙G + G 2 = 0 ∆k = G k - is the incident wave vector k’ - is the reflected wave vector G - is a reciprocal lattice vector such that where G = ∆k = k - k’ the diffraction condition is met
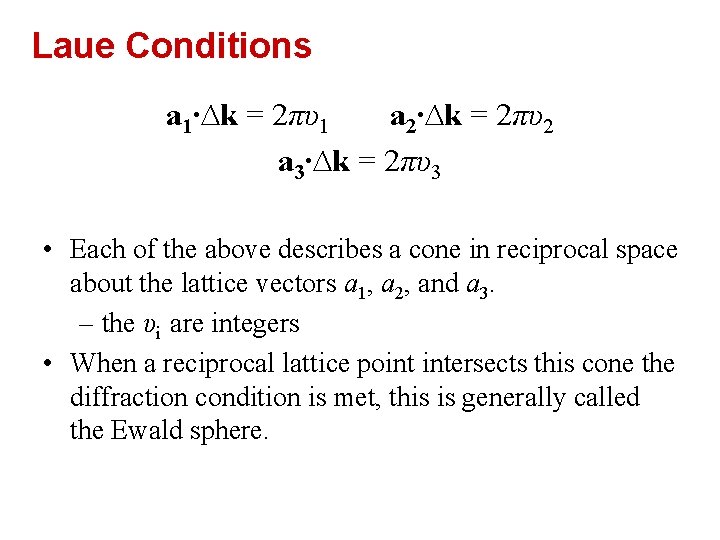
Laue Conditions a 1∙∆k = 2πυ1 a 2∙∆k = 2πυ2 a 3∙∆k = 2πυ3 • Each of the above describes a cone in reciprocal space about the lattice vectors a 1, a 2, and a 3. – the υi are integers • When a reciprocal lattice point intersects this cone the diffraction condition is met, this is generally called the Ewald sphere.
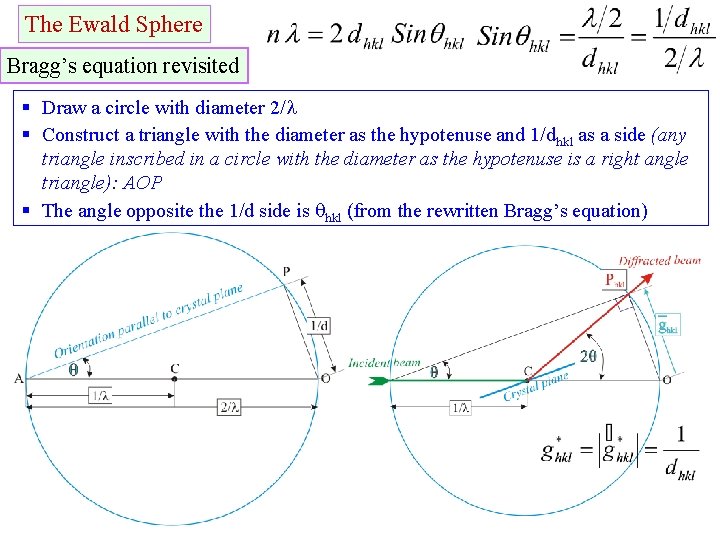
The Ewald Sphere Bragg’s equation revisited § Draw a circle with diameter 2/ § Construct a triangle with the diameter as the hypotenuse and 1/dhkl as a side (any triangle inscribed in a circle with the diameter as the hypotenuse is a right angle triangle): AOP § The angle opposite the 1/d side is hkl (from the rewritten Bragg’s equation)
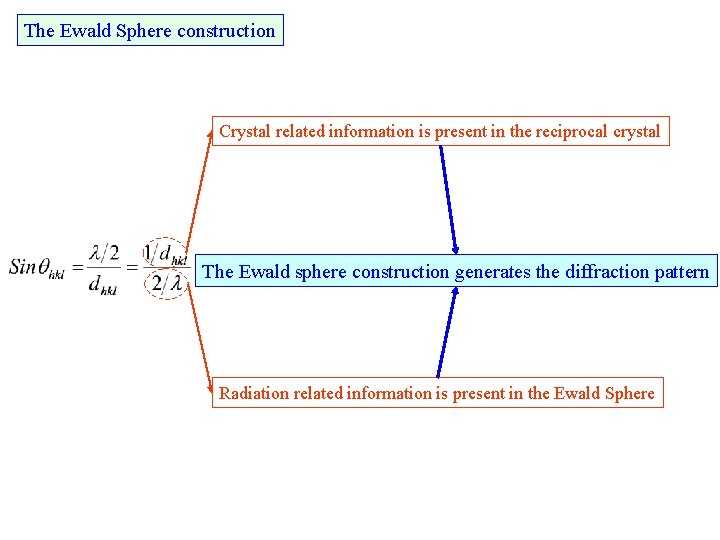
The Ewald Sphere construction Crystal related information is present in the reciprocal crystal The Ewald sphere construction generates the diffraction pattern Radiation related information is present in the Ewald Sphere
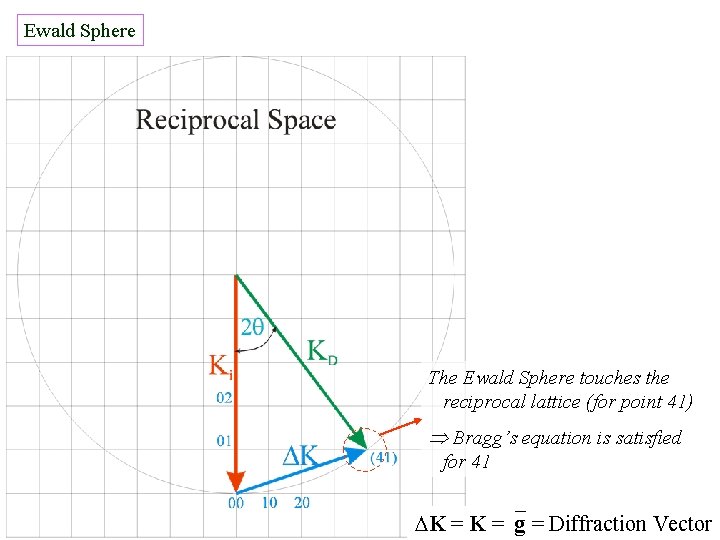
Ewald Sphere The Ewald Sphere touches the reciprocal lattice (for point 41) Bragg’s equation is satisfied for 41 K = g = Diffraction Vector
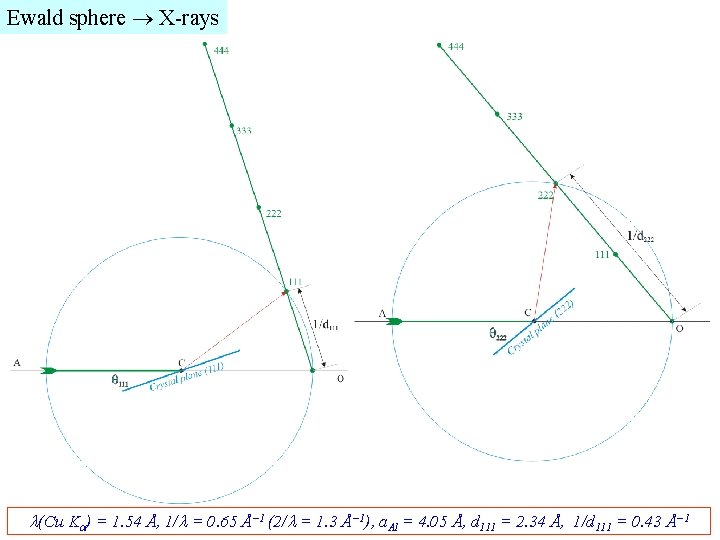
Ewald sphere X-rays (Cu K ) = 1. 54 Å, 1/ = 0. 65 Å− 1 (2/ = 1. 3 Å− 1), a. Al = 4. 05 Å, d 111 = 2. 34 Å, 1/d 111 = 0. 43 Å− 1
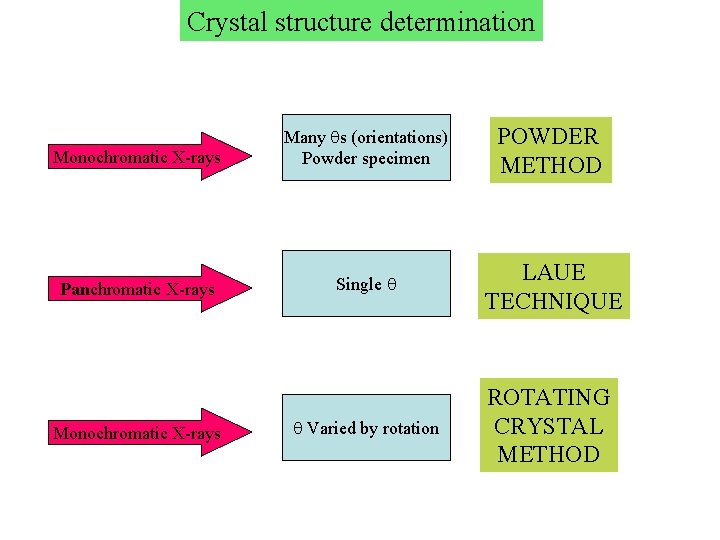
Crystal structure determination Monochromatic X-rays Many s (orientations) Powder specimen POWDER METHOD Panchromatic X-rays Single LAUE TECHNIQUE Monochromatic X-rays Varied by rotation ROTATING CRYSTAL METHOD
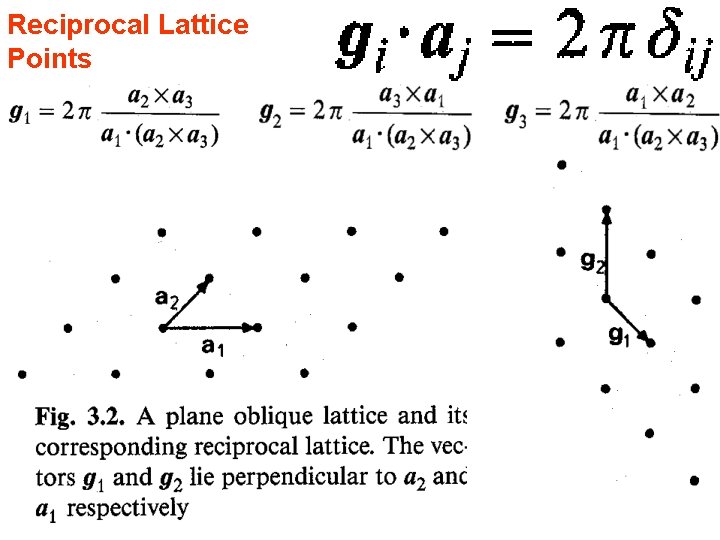
Reciprocal Lattice Points
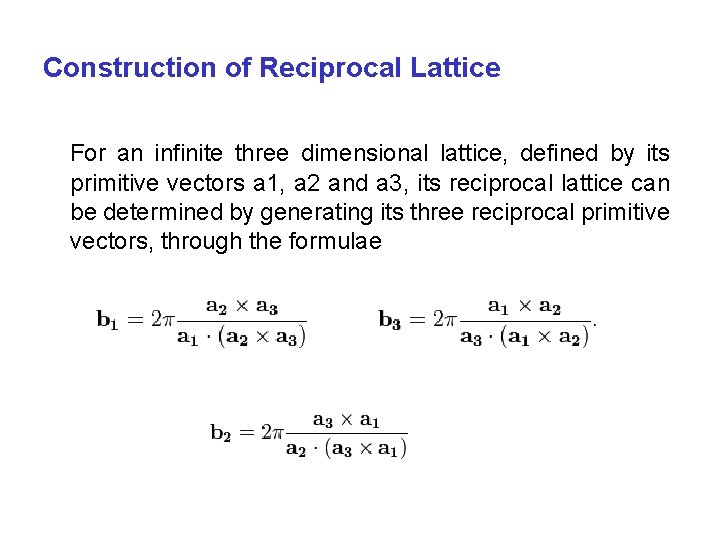
Construction of Reciprocal Lattice For an infinite three dimensional lattice, defined by its primitive vectors a 1, a 2 and a 3, its reciprocal lattice can be determined by generating its three reciprocal primitive vectors, through the formulae
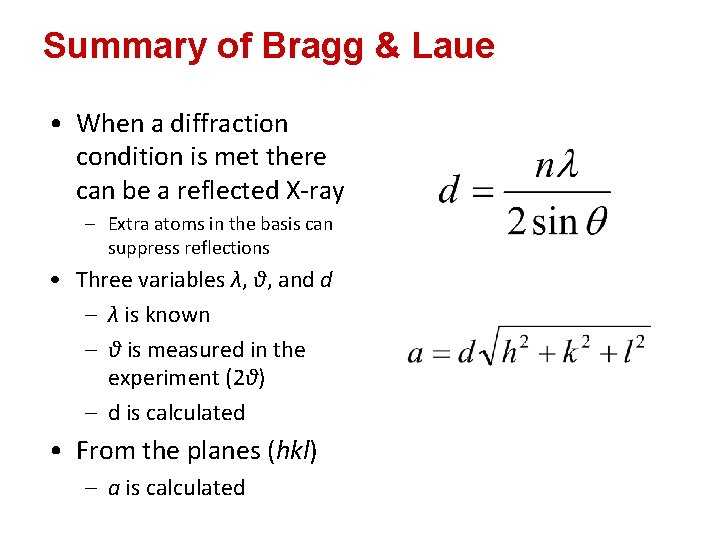
Summary of Bragg & Laue • When a diffraction condition is met there can be a reflected X-ray – Extra atoms in the basis can suppress reflections • Three variables λ, θ, and d – λ is known – θ is measured in the experiment (2θ) – d is calculated • From the planes (hkl) – a is calculated
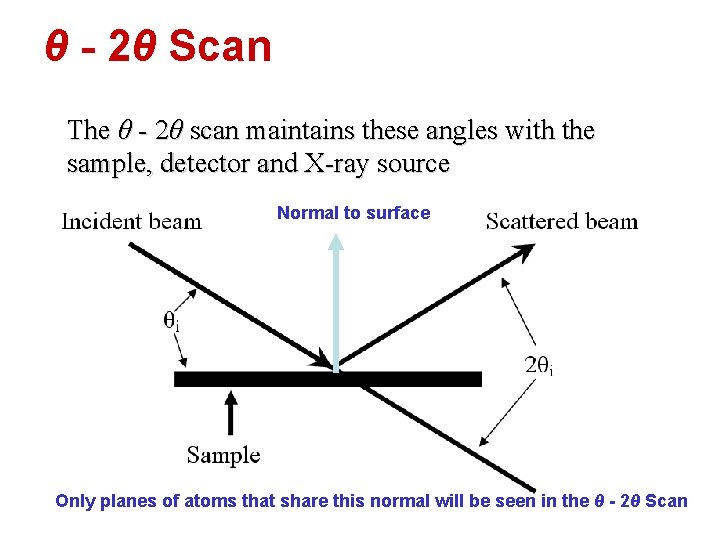
θ - 2θ Scan The θ - 2θ scan maintains these angles with the sample, detector and X-ray source Normal to surface Only planes of atoms that share this normal will be seen in the θ - 2θ Scan
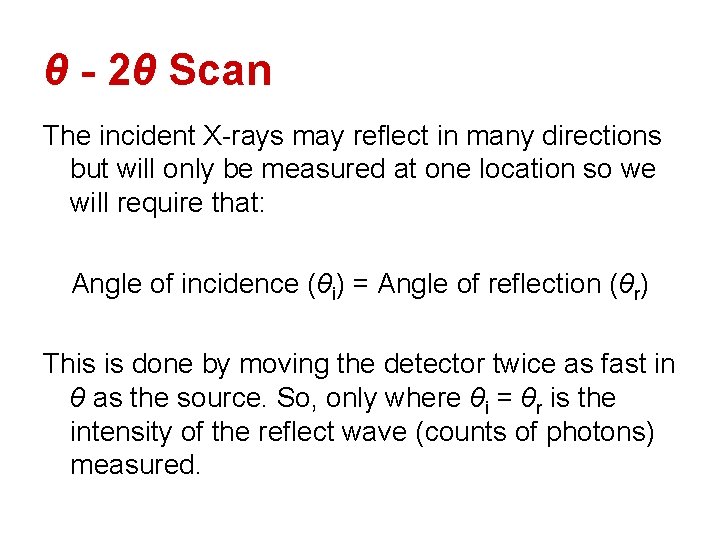
θ - 2θ Scan The incident X-rays may reflect in many directions but will only be measured at one location so we will require that: Angle of incidence (θi) = Angle of reflection (θr) This is done by moving the detector twice as fast in θ as the source. So, only where θi = θr is the intensity of the reflect wave (counts of photons) measured.
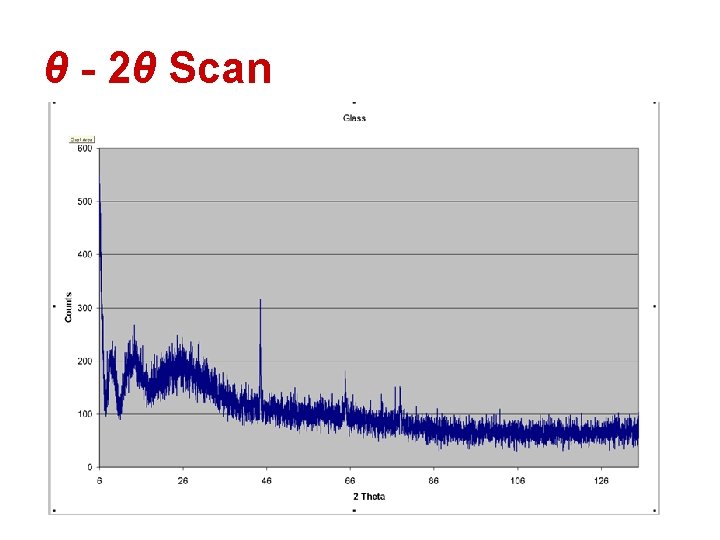
θ - 2θ Scan
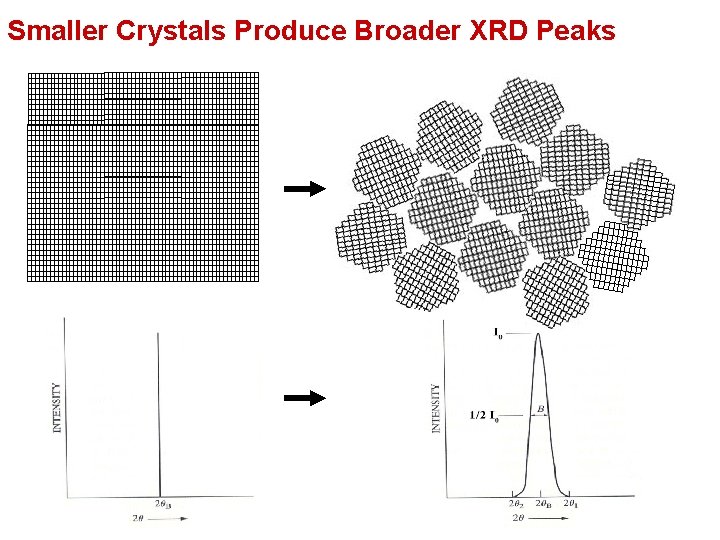
Smaller Crystals Produce Broader XRD Peaks
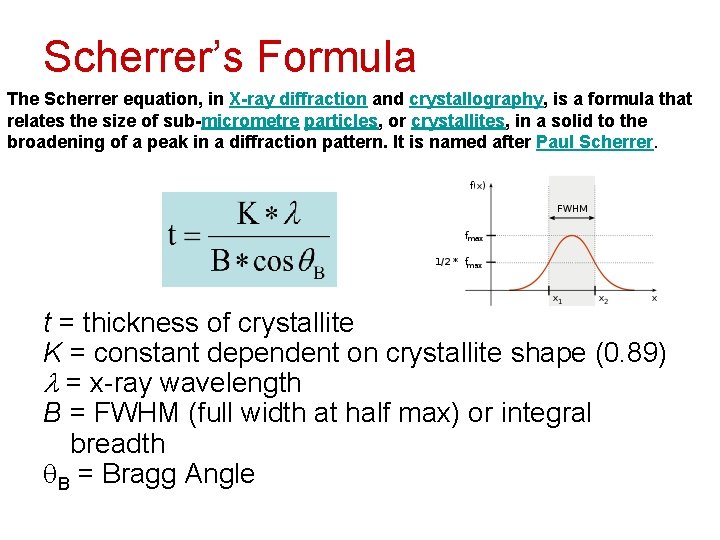
Scherrer’s Formula The Scherrer equation, in X-ray diffraction and crystallography, is a formula that relates the size of sub-micrometre particles, or crystallites, in a solid to the broadening of a peak in a diffraction pattern. It is named after Paul Scherrer. t = thickness of crystallite K = constant dependent on crystallite shape (0. 89) = x-ray wavelength B = FWHM (full width at half max) or integral breadth B = Bragg Angle
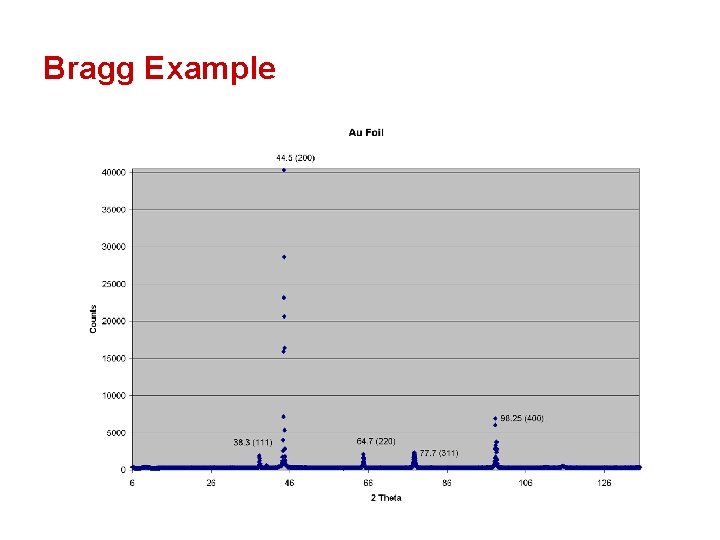
Bragg Example
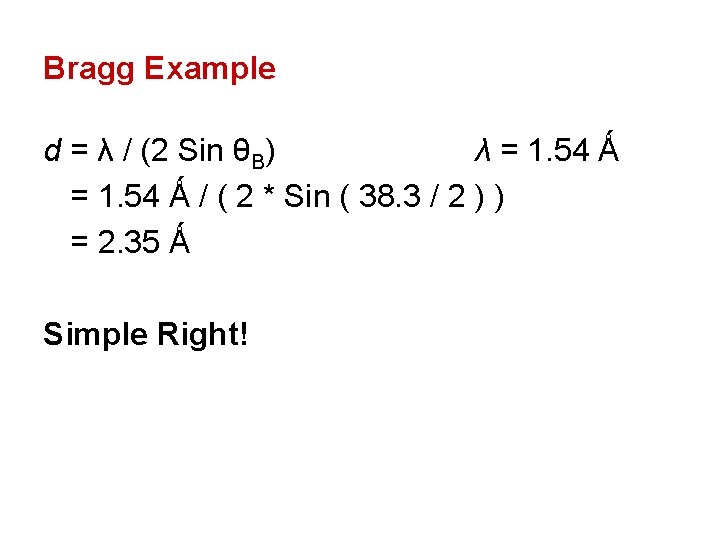
Bragg Example d = λ / (2 Sin θB) λ = 1. 54 Ǻ / ( 2 * Sin ( 38. 3 / 2 ) ) = 2. 35 Ǻ Simple Right!
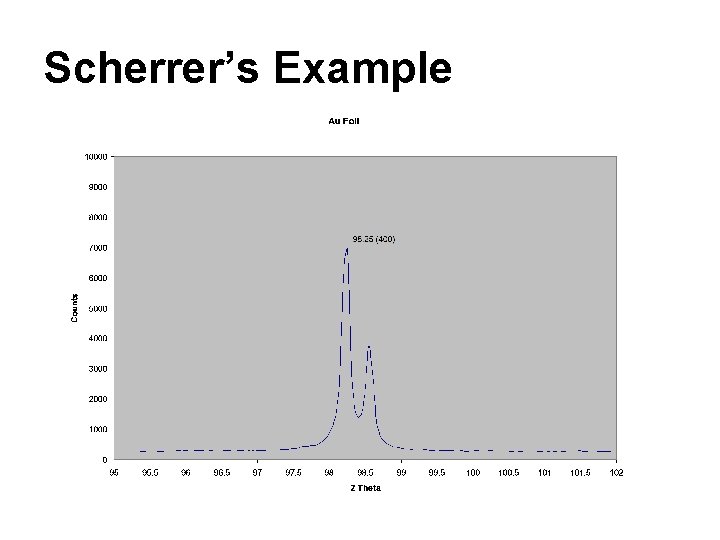
Scherrer’s Example
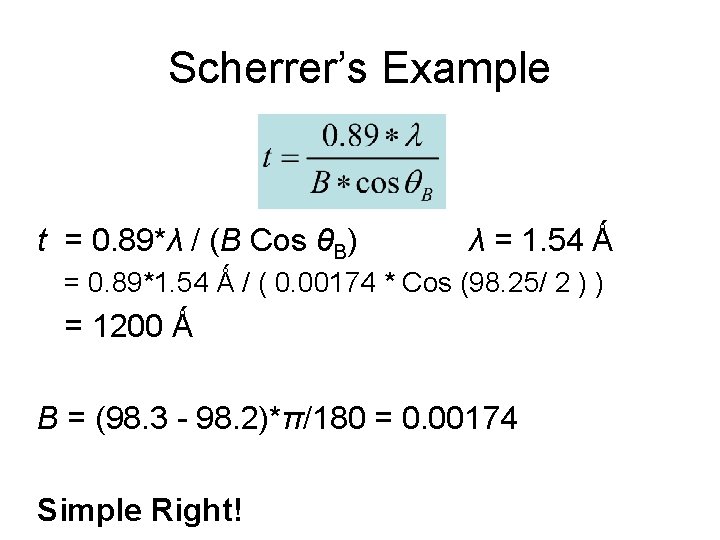
Scherrer’s Example t = 0. 89*λ / (B Cos θB) λ = 1. 54 Ǻ = 0. 89*1. 54 Ǻ / ( 0. 00174 * Cos (98. 25/ 2 ) ) = 1200 Ǻ B = (98. 3 - 98. 2)*π/180 = 0. 00174 Simple Right!
Insidan region jh
L a t t i c e
Bcc structure factor
Bragg's law in reciprocal lattice formula
Laue equation
Reciprocal lattice
Brillouin zone
Reciprocal metric tensor
Reciprocal lattice
Ewald construction in reciprocal lattice
Ngoại tâm thu thất chùm đôi
Block xoang nhĩ
Thơ thất ngôn tứ tuyệt đường luật
Thơ thất ngôn tứ tuyệt đường luật
Chiến lược kinh doanh quốc tế của walmart
Tìm vết của đường thẳng
Hãy nói thật ít để làm được nhiều
Tôn thất thuyết là ai
Gây tê cơ vuông thắt lưng
Sau thất bại ở hồ điển triệt
Partially addressed mail
Example of non programmed decision
The third epistle of st. john is addressed to________.
Partially addressed mail service
Essential elements of a contract
Technology addressed
U types
Invoked audience
Chapter 20 today's issues in africa
Ethical and legal issues chapter 2