Chapter 10 Applied Thermodynamics for Engineering Technologists Eastop
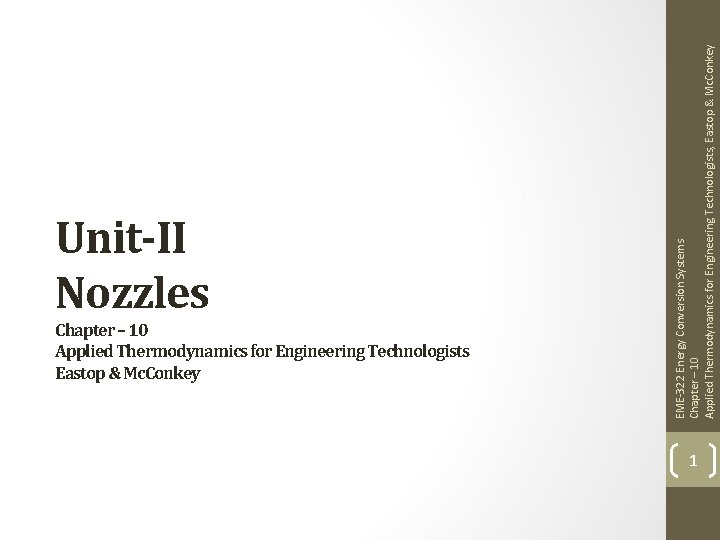
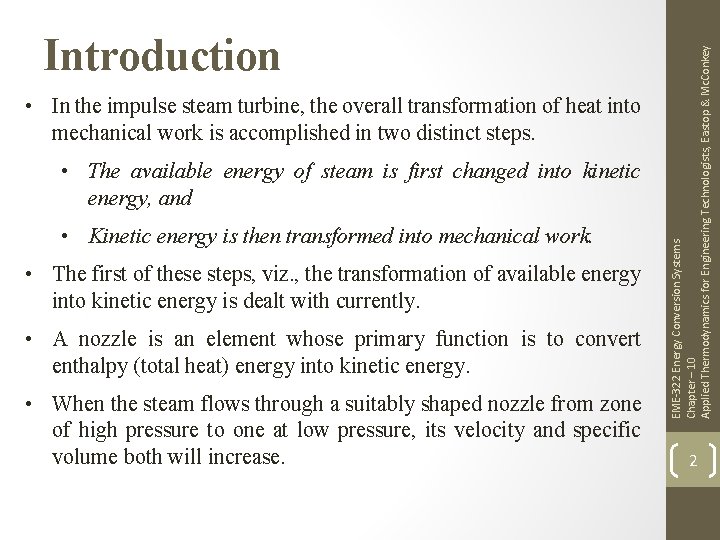
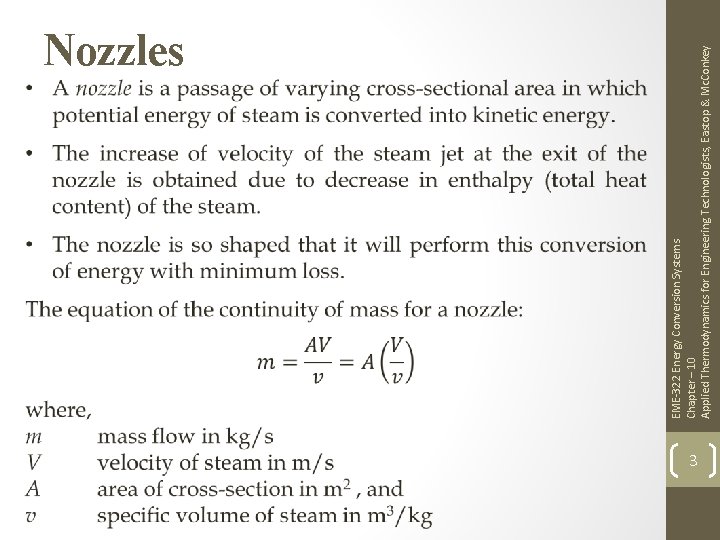
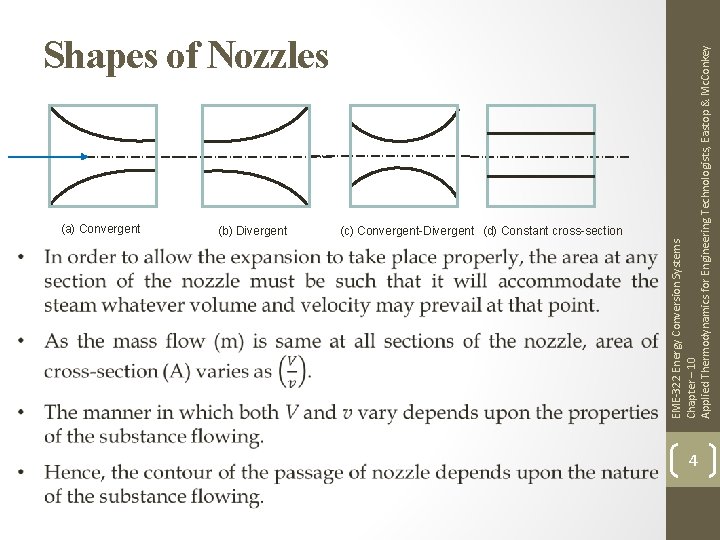
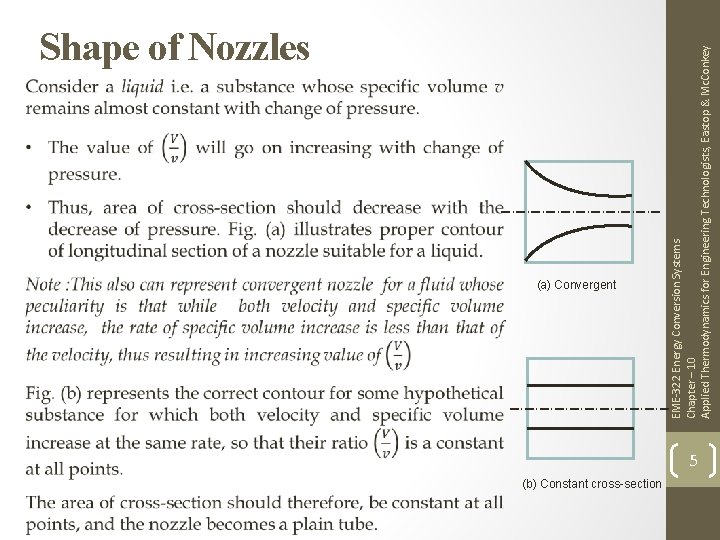
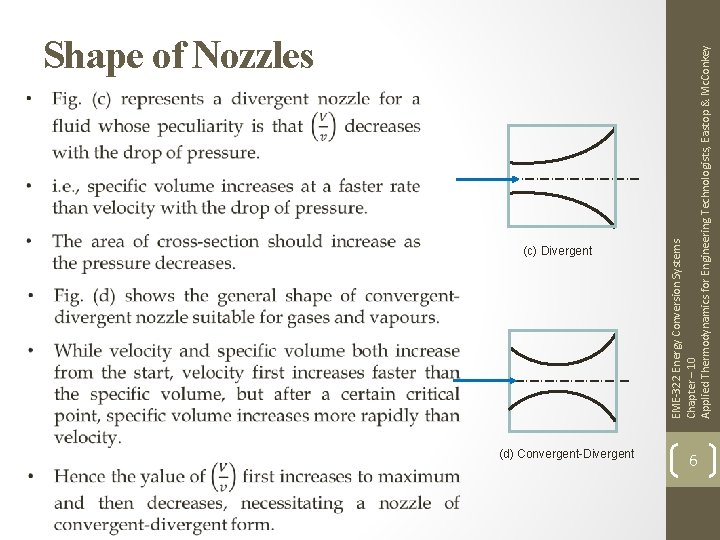
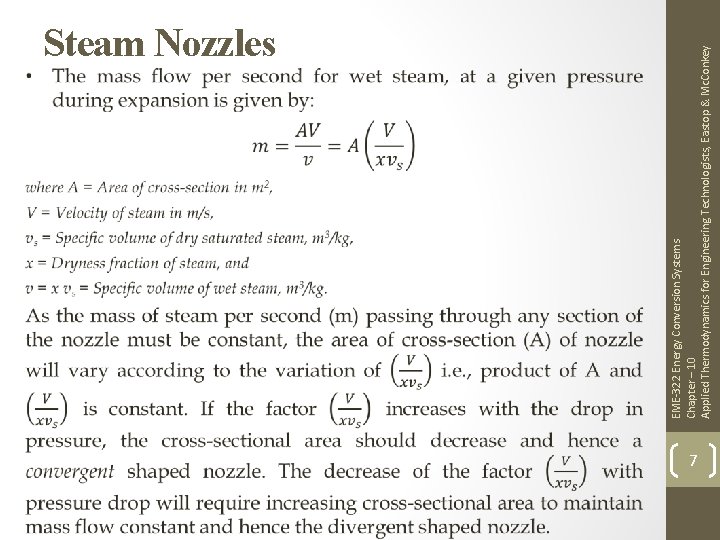
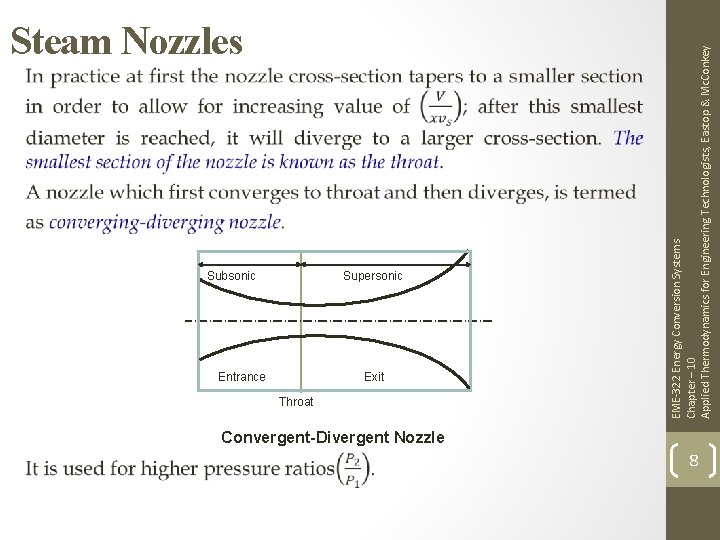
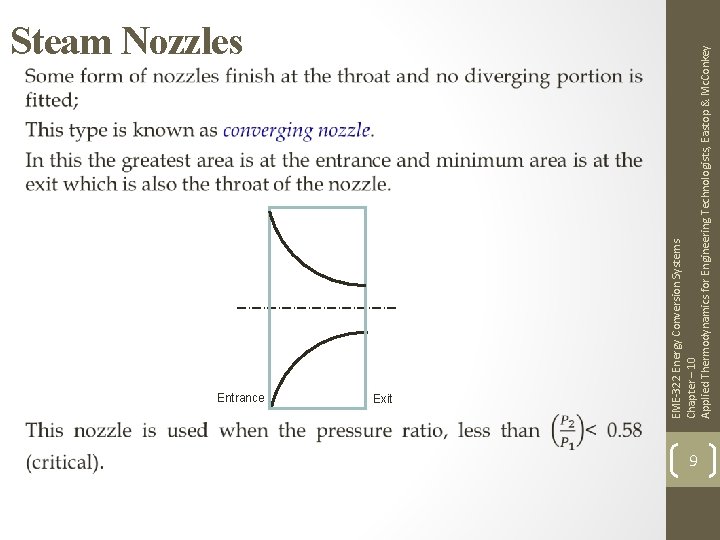
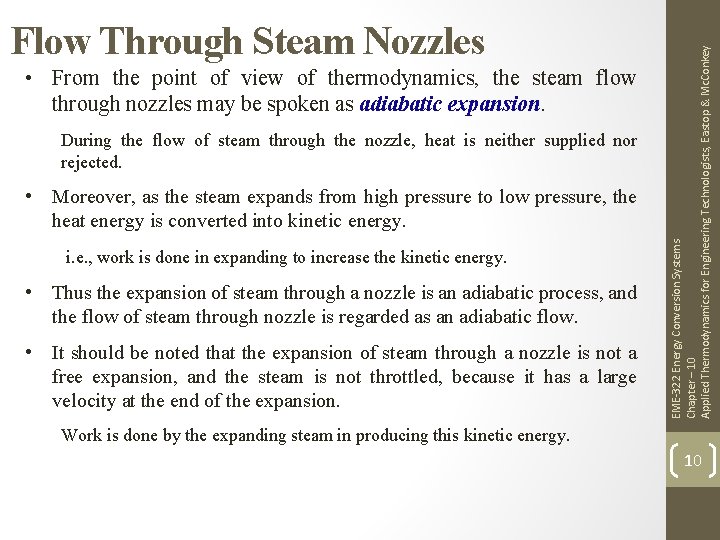
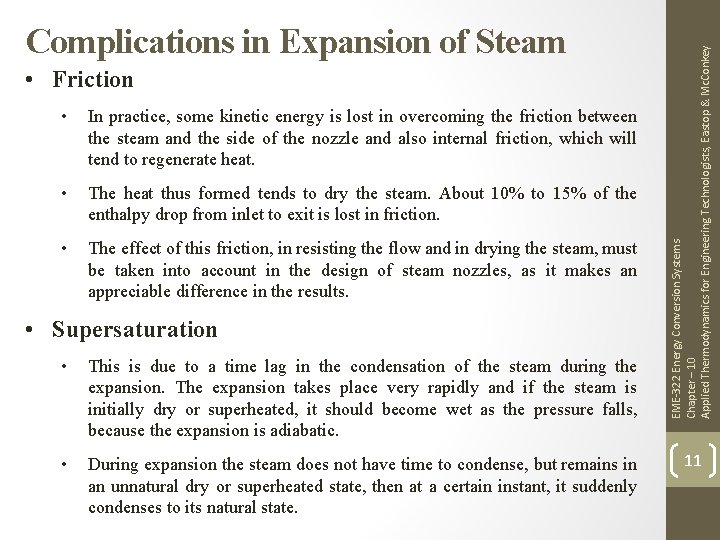
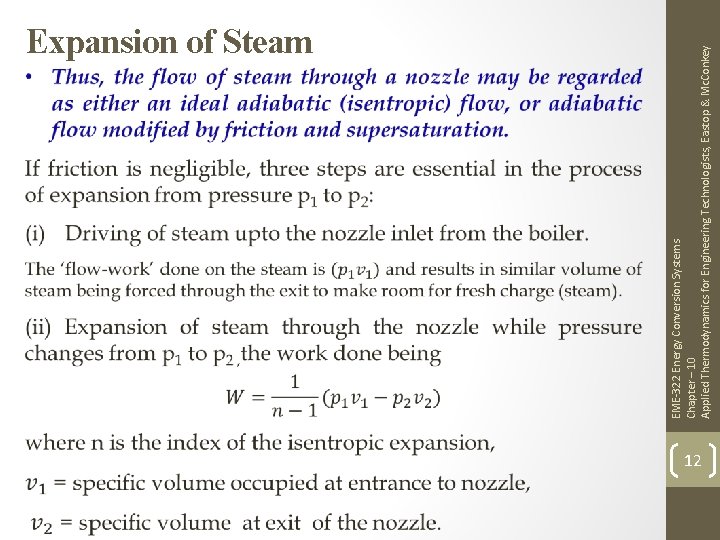
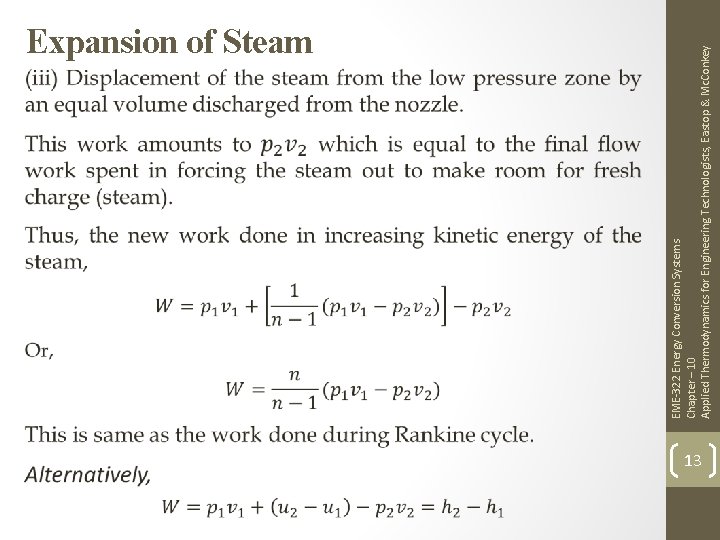
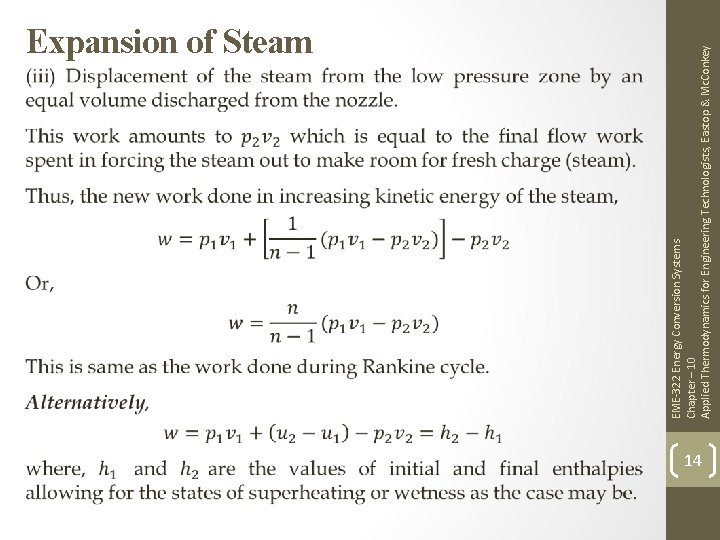
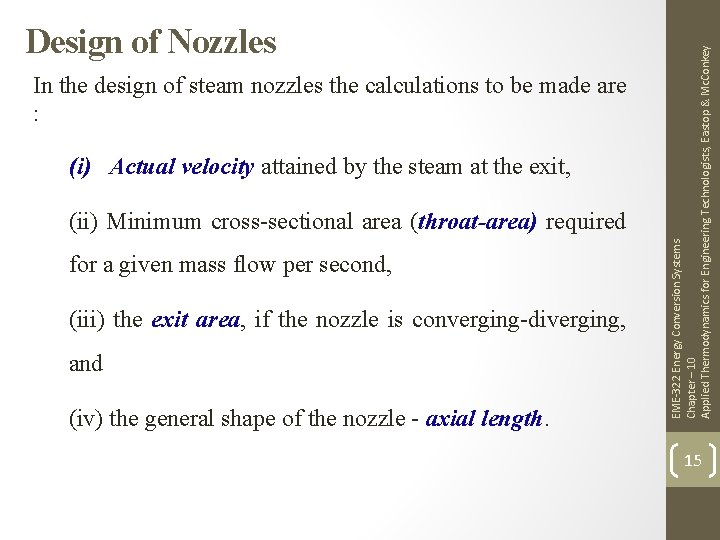
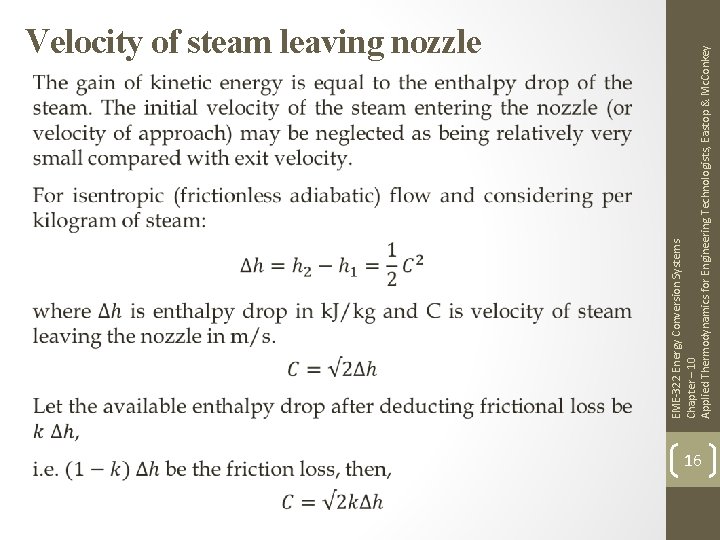
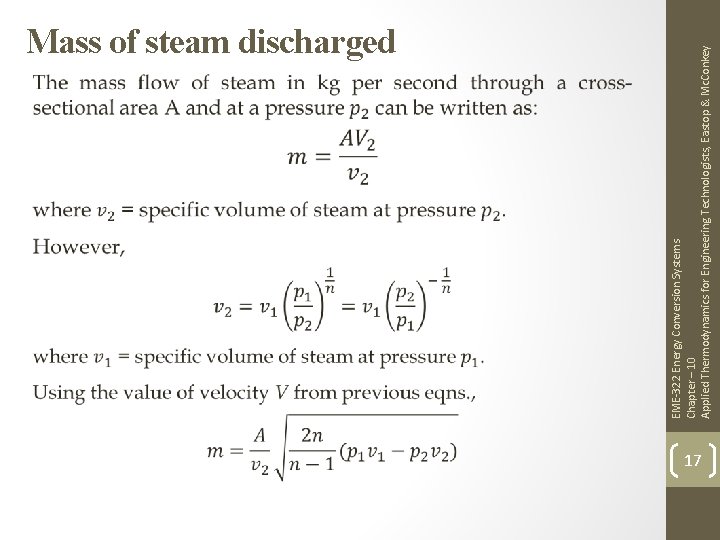
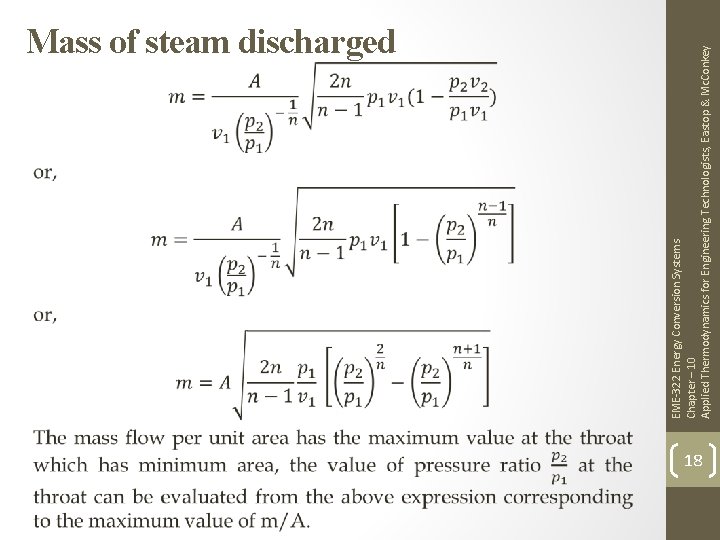
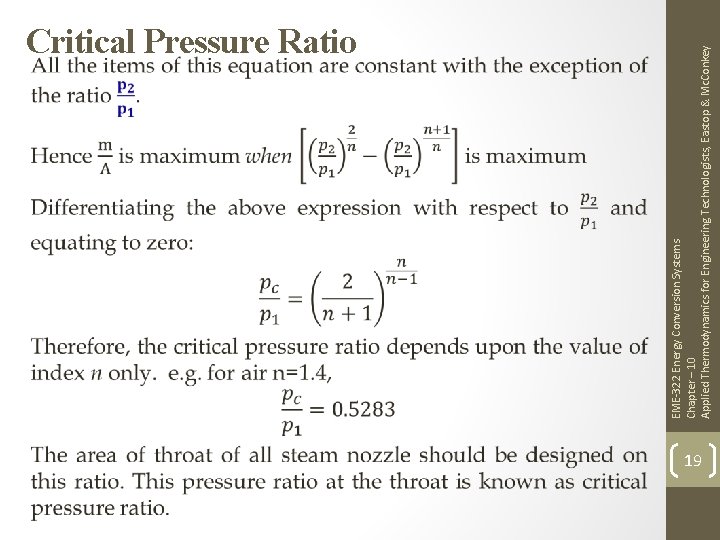
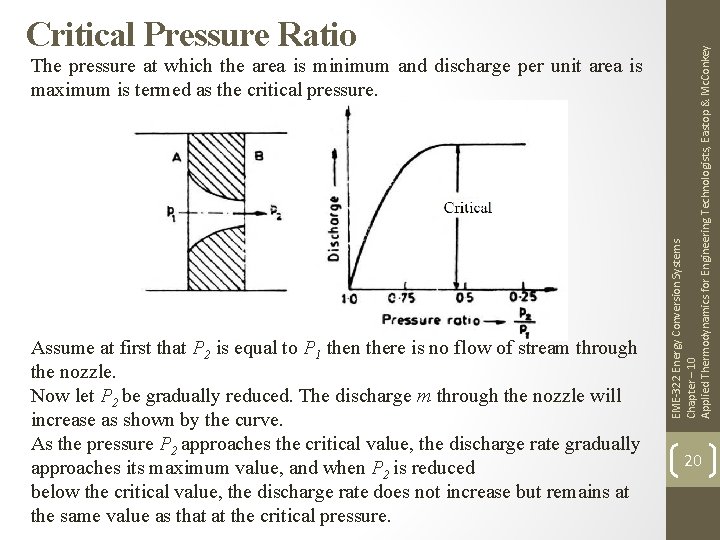
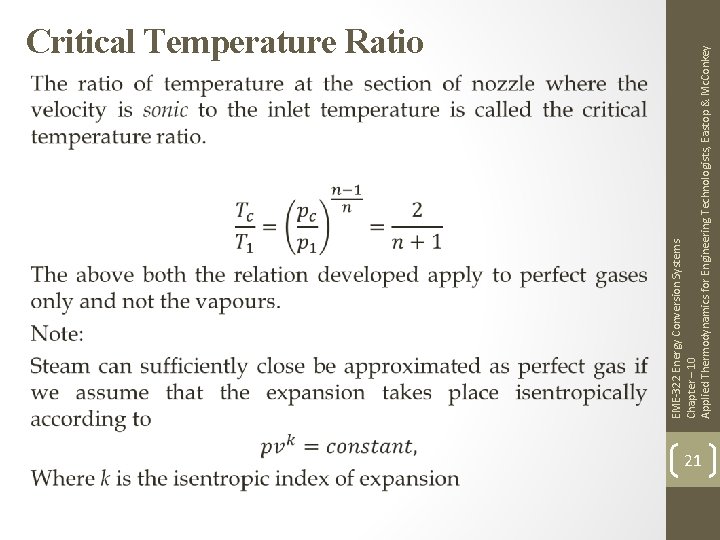
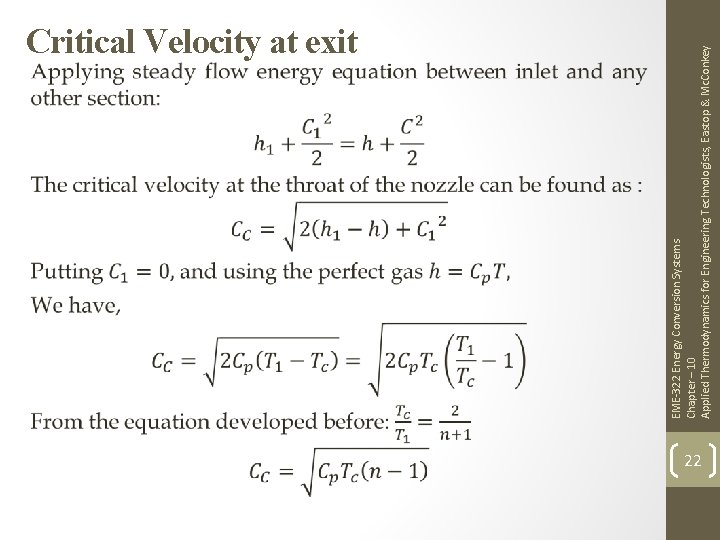
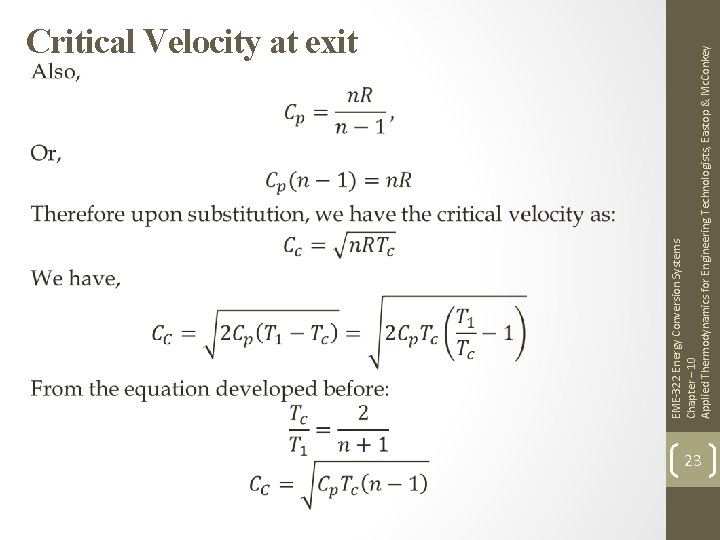
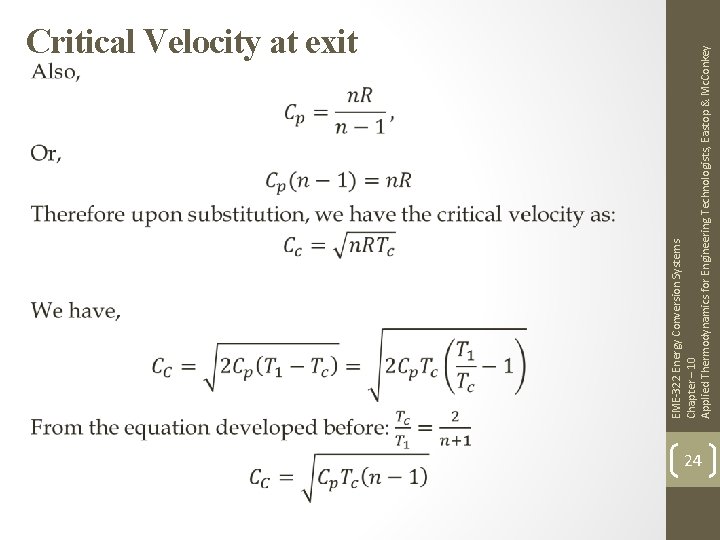
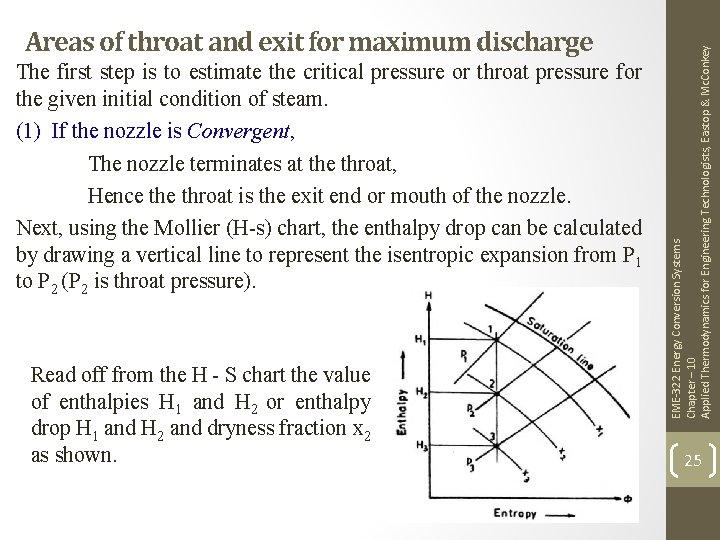
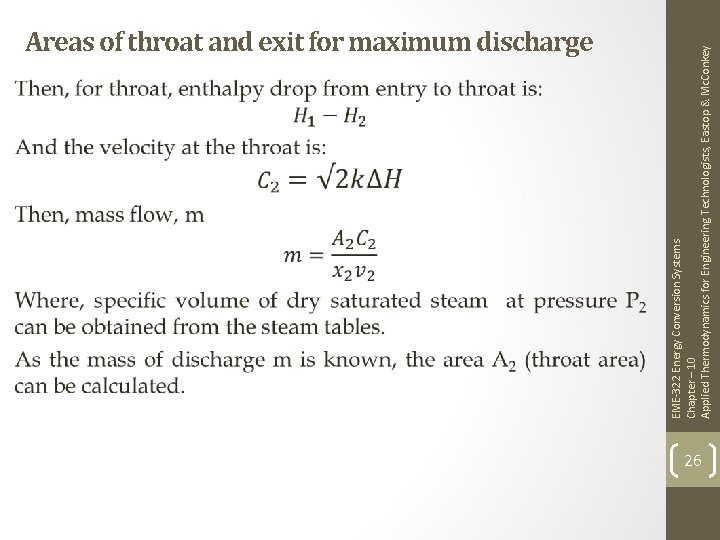
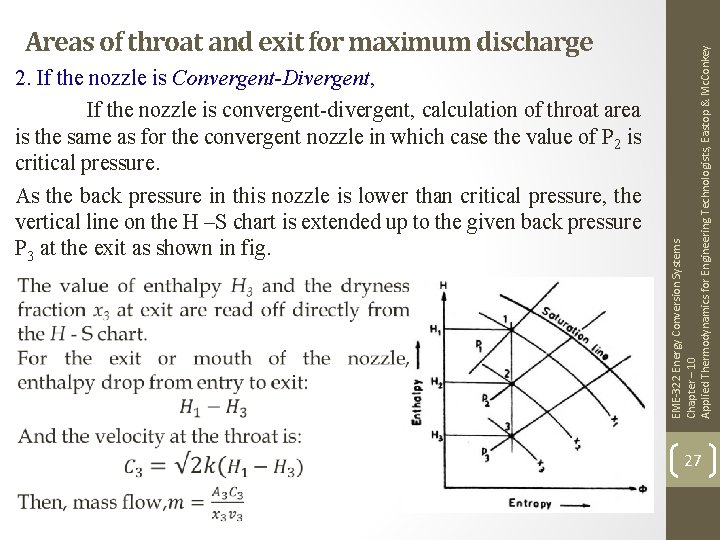
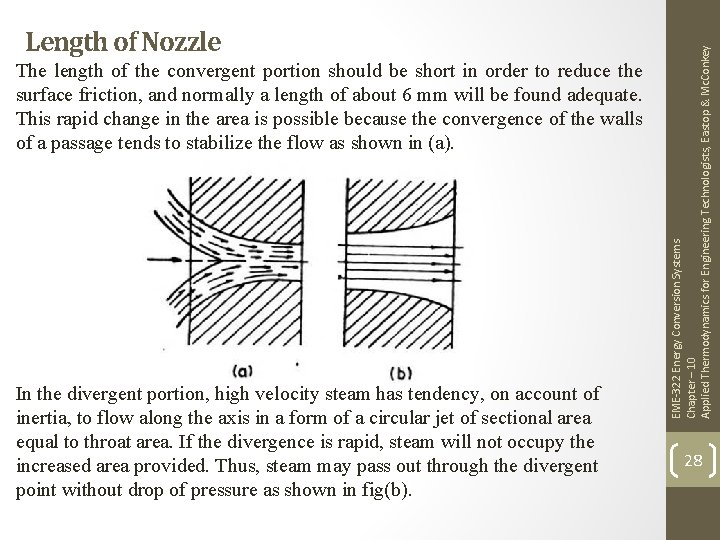
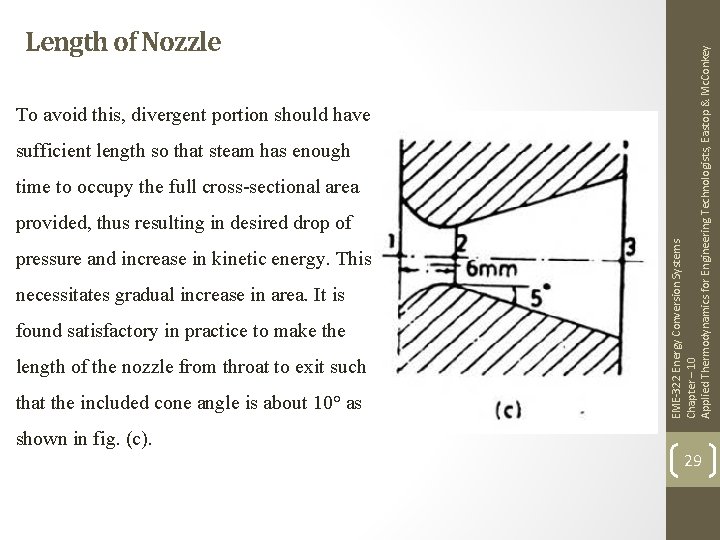
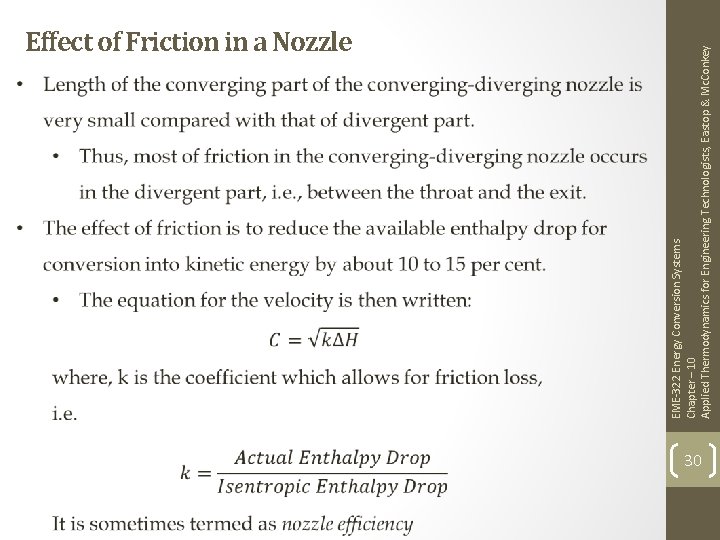
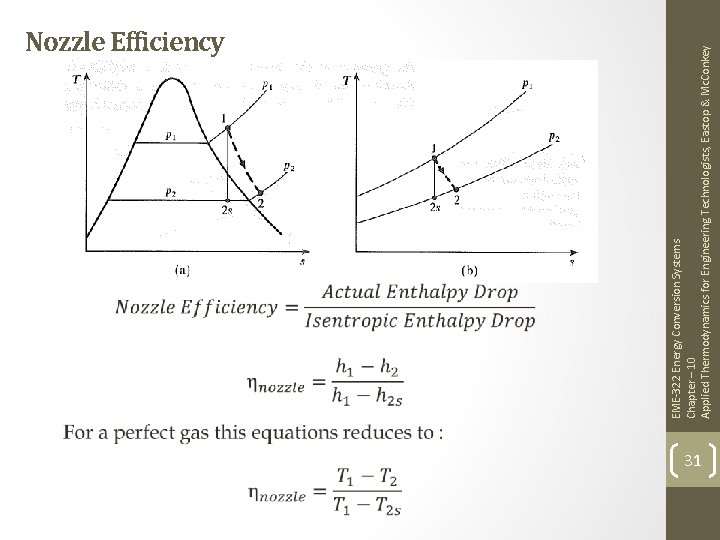
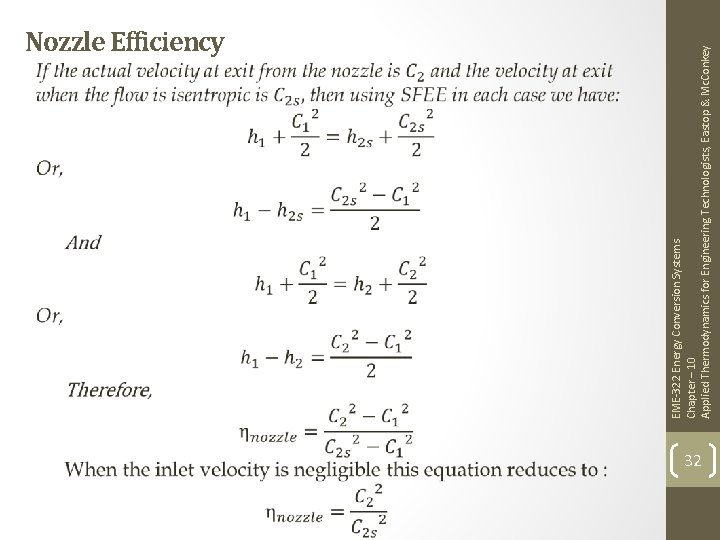
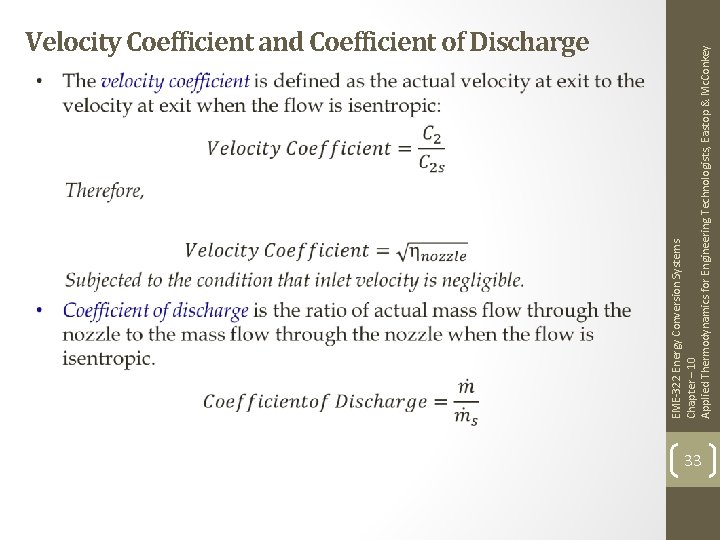
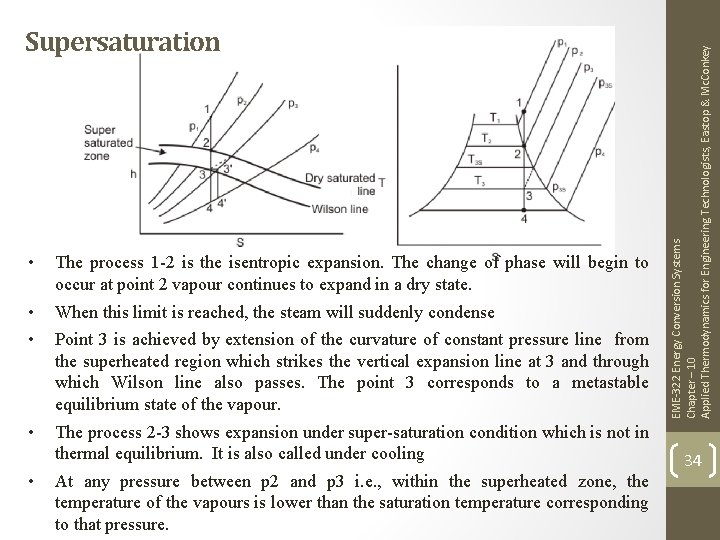
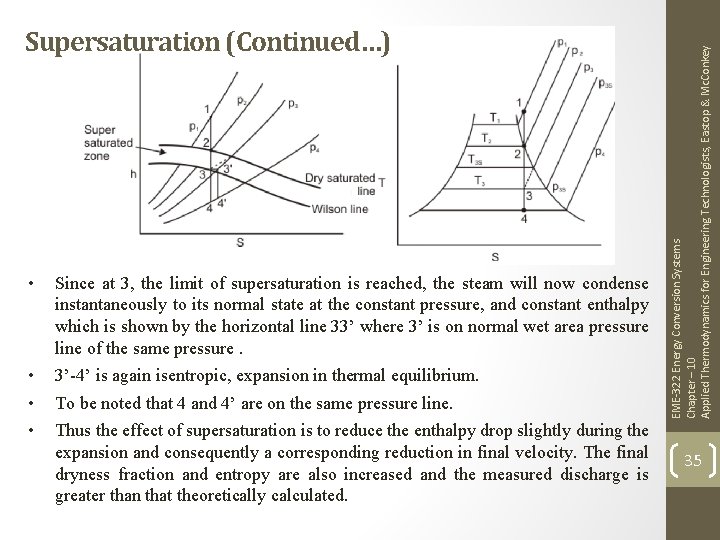
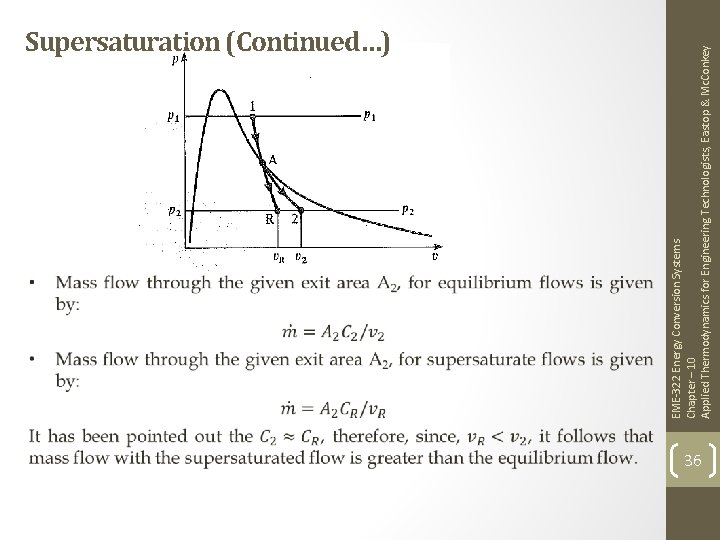
- Slides: 36
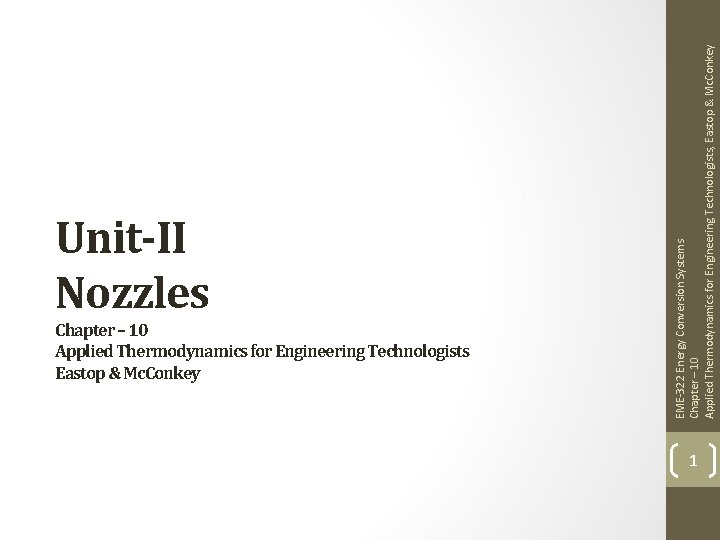
Chapter – 10 Applied Thermodynamics for Engineering Technologists Eastop & Mc. Conkey EME-322 Energy Conversion Systems Chapter – 10 Applied Thermodynamics for Engineering Technologists, Eastop & Mc. Conkey Unit-II Nozzles 1
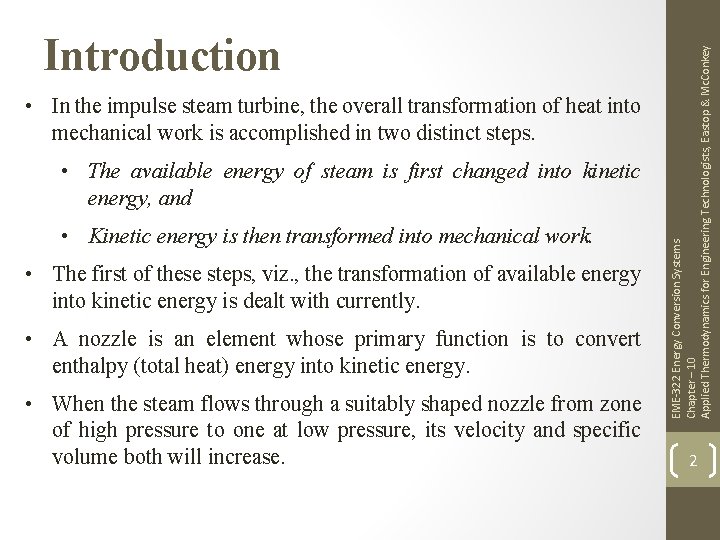
• In the impulse steam turbine, the overall transformation of heat into mechanical work is accomplished in two distinct steps. • The available energy of steam is first changed into kinetic energy, and • Kinetic energy is then transformed into mechanical work. • The first of these steps, viz. , the transformation of available energy into kinetic energy is dealt with currently. • A nozzle is an element whose primary function is to convert enthalpy (total heat) energy into kinetic energy. • When the steam flows through a suitably shaped nozzle from zone of high pressure to one at low pressure, its velocity and specific volume both will increase. EME-322 Energy Conversion Systems Chapter – 10 Applied Thermodynamics for Engineering Technologists, Eastop & Mc. Conkey Introduction 2
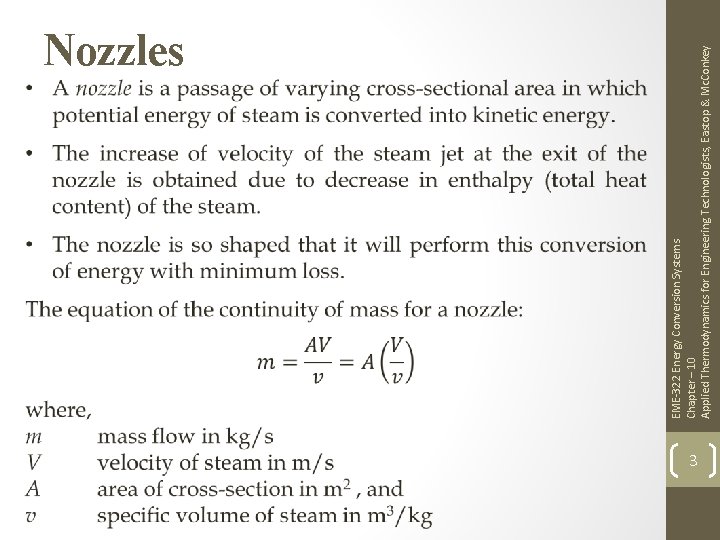
EME-322 Energy Conversion Systems Chapter – 10 Applied Thermodynamics for Engineering Technologists, Eastop & Mc. Conkey Nozzles 3
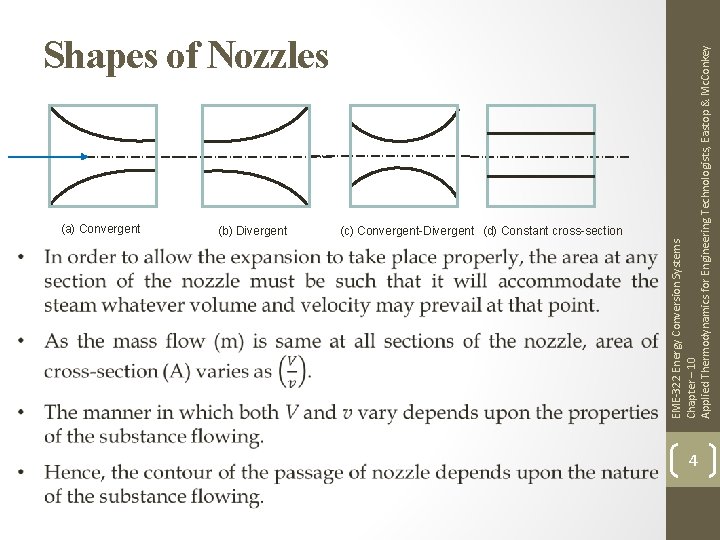
(a) Convergent (b) Divergent (c) Convergent-Divergent (d) Constant cross-section EME-322 Energy Conversion Systems Chapter – 10 Applied Thermodynamics for Engineering Technologists, Eastop & Mc. Conkey Shapes of Nozzles 4
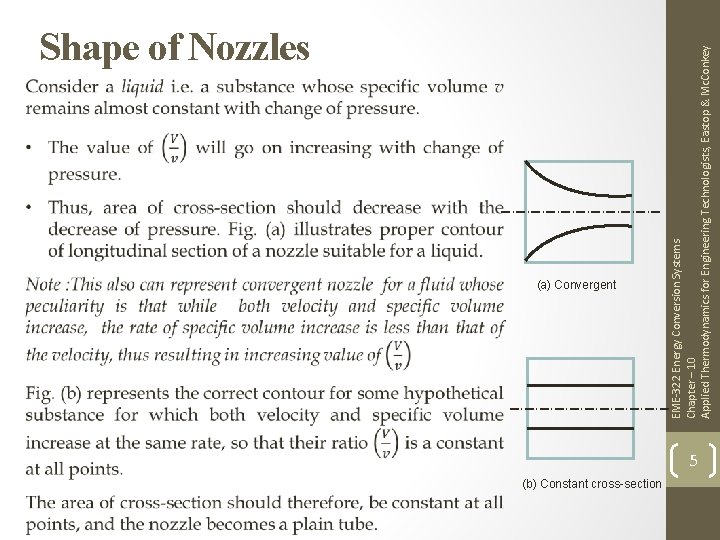
(a) Convergent (b) Constant cross-section EME-322 Energy Conversion Systems Chapter – 10 Applied Thermodynamics for Engineering Technologists, Eastop & Mc. Conkey Shape of Nozzles 5
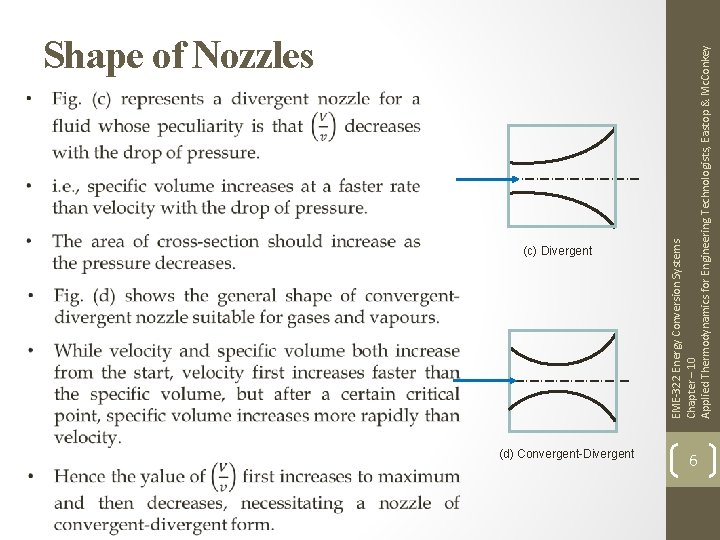
(c) Divergent (d) Convergent-Divergent EME-322 Energy Conversion Systems Chapter – 10 Applied Thermodynamics for Engineering Technologists, Eastop & Mc. Conkey Shape of Nozzles 6
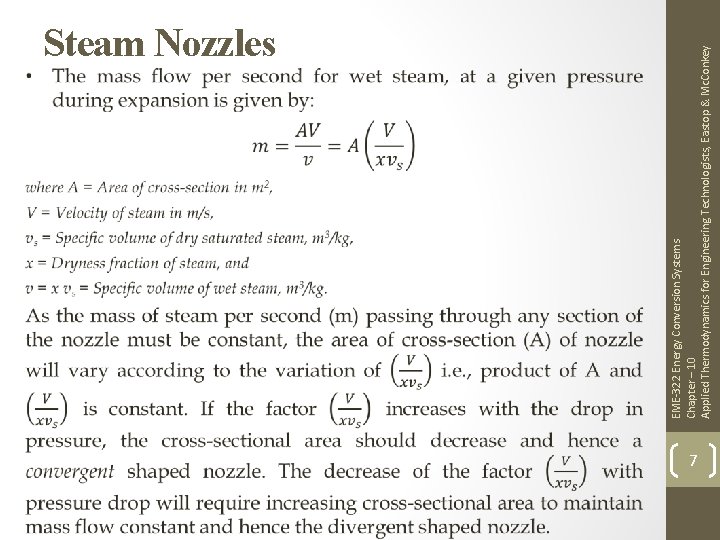
EME-322 Energy Conversion Systems Chapter – 10 Applied Thermodynamics for Engineering Technologists, Eastop & Mc. Conkey Steam Nozzles 7
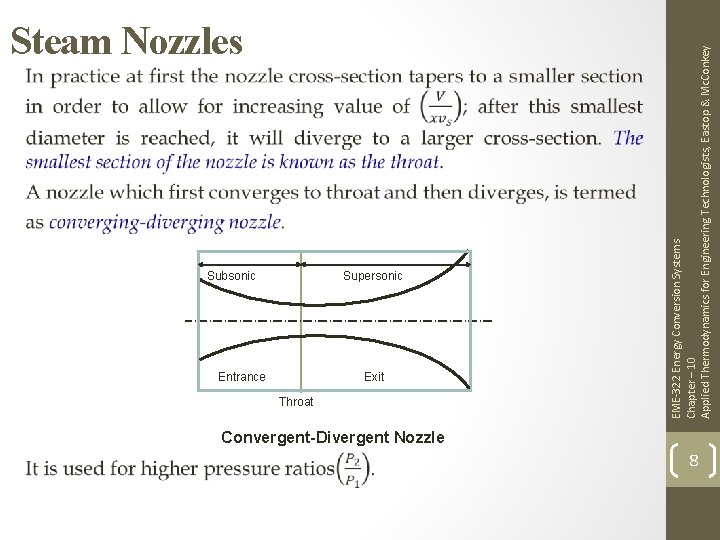
Subsonic Supersonic Entrance Exit Throat EME-322 Energy Conversion Systems Chapter – 10 Applied Thermodynamics for Engineering Technologists, Eastop & Mc. Conkey Steam Nozzles Convergent-Divergent Nozzle 8
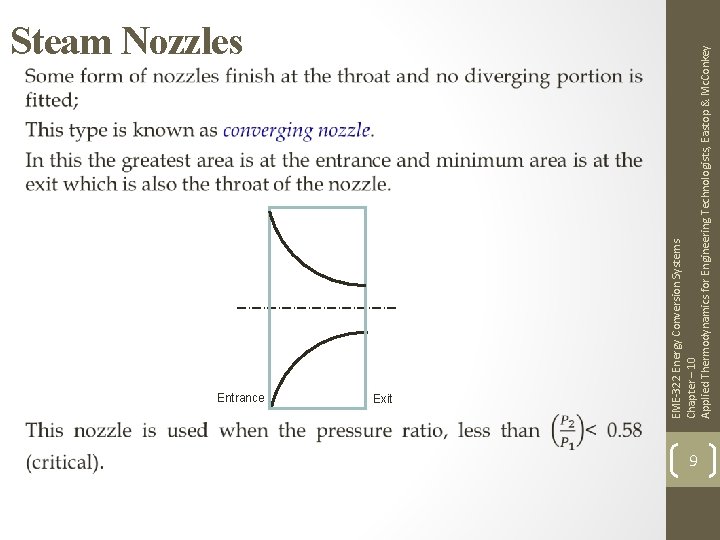
Entrance Exit EME-322 Energy Conversion Systems Chapter – 10 Applied Thermodynamics for Engineering Technologists, Eastop & Mc. Conkey Steam Nozzles 9
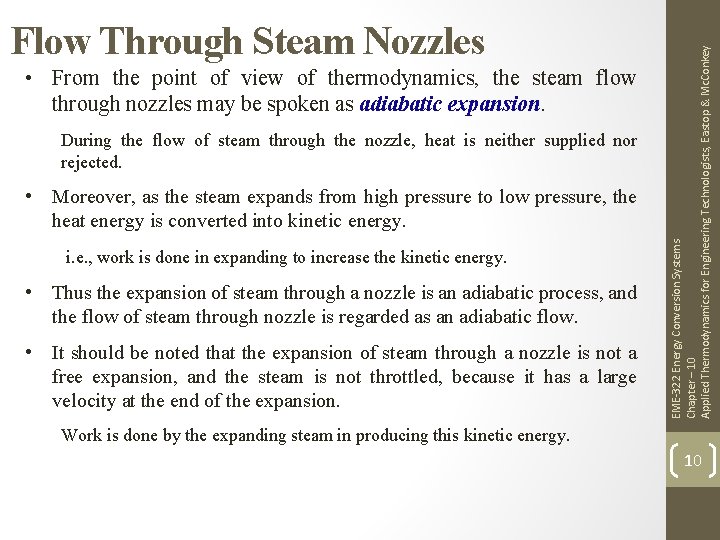
• From the point of view of thermodynamics, the steam flow through nozzles may be spoken as adiabatic expansion. During the flow of steam through the nozzle, heat is neither supplied nor rejected. • Moreover, as the steam expands from high pressure to low pressure, the heat energy is converted into kinetic energy. i. e. , work is done in expanding to increase the kinetic energy. • Thus the expansion of steam through a nozzle is an adiabatic process, and the flow of steam through nozzle is regarded as an adiabatic flow. • It should be noted that the expansion of steam through a nozzle is not a free expansion, and the steam is not throttled, because it has a large velocity at the end of the expansion. EME-322 Energy Conversion Systems Chapter – 10 Applied Thermodynamics for Engineering Technologists, Eastop & Mc. Conkey Flow Through Steam Nozzles Work is done by the expanding steam in producing this kinetic energy. 10
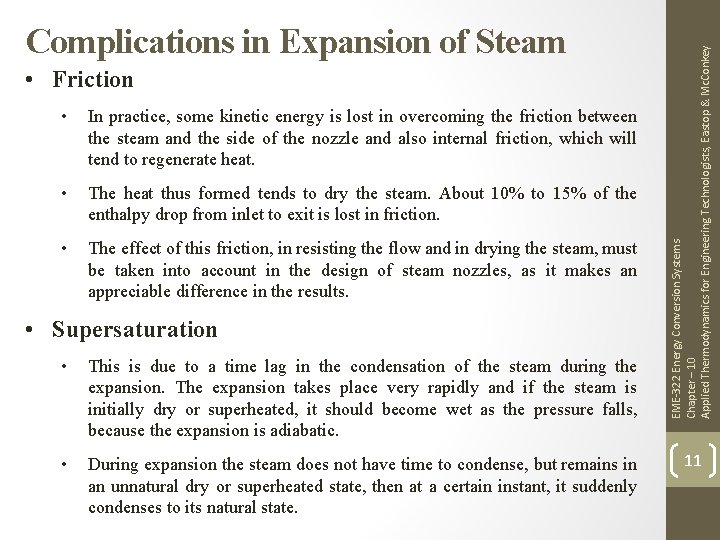
• Friction • In practice, some kinetic energy is lost in overcoming the friction between the steam and the side of the nozzle and also internal friction, which will tend to regenerate heat. • The heat thus formed tends to dry the steam. About 10% to 15% of the enthalpy drop from inlet to exit is lost in friction. • The effect of this friction, in resisting the flow and in drying the steam, must be taken into account in the design of steam nozzles, as it makes an appreciable difference in the results. • Supersaturation • This is due to a time lag in the condensation of the steam during the expansion. The expansion takes place very rapidly and if the steam is initially dry or superheated, it should become wet as the pressure falls, because the expansion is adiabatic. • During expansion the steam does not have time to condense, but remains in an unnatural dry or superheated state, then at a certain instant, it suddenly condenses to its natural state. EME-322 Energy Conversion Systems Chapter – 10 Applied Thermodynamics for Engineering Technologists, Eastop & Mc. Conkey Complications in Expansion of Steam 11
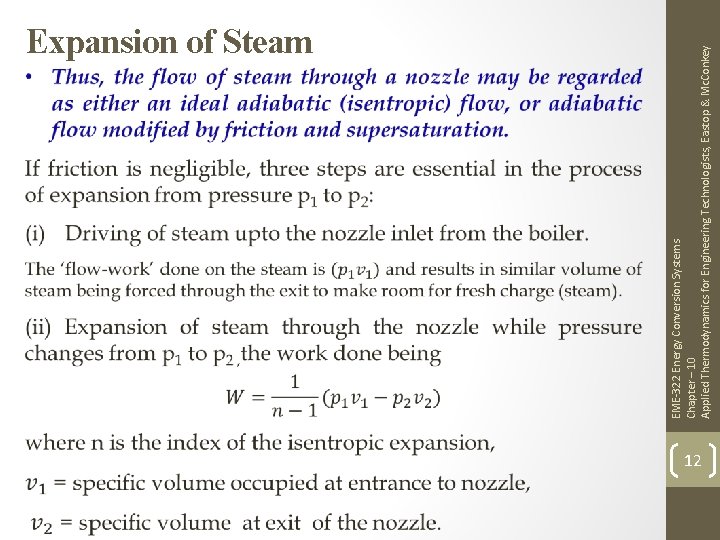
EME-322 Energy Conversion Systems Chapter – 10 Applied Thermodynamics for Engineering Technologists, Eastop & Mc. Conkey Expansion of Steam 12
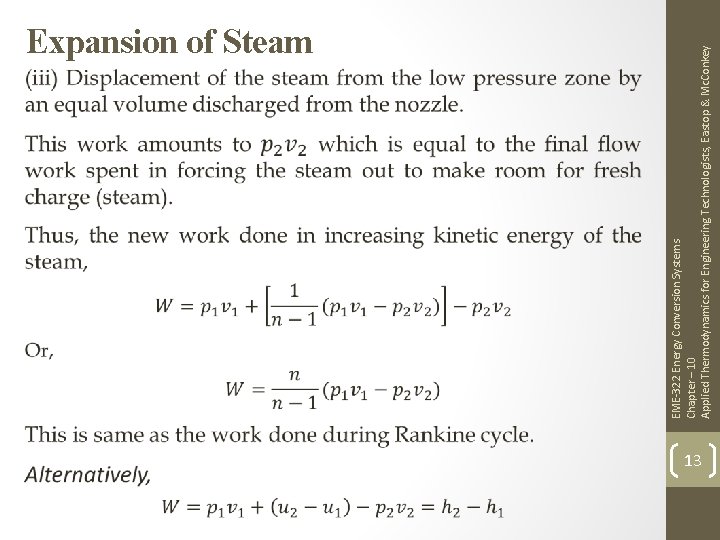
EME-322 Energy Conversion Systems Chapter – 10 Applied Thermodynamics for Engineering Technologists, Eastop & Mc. Conkey Expansion of Steam 13
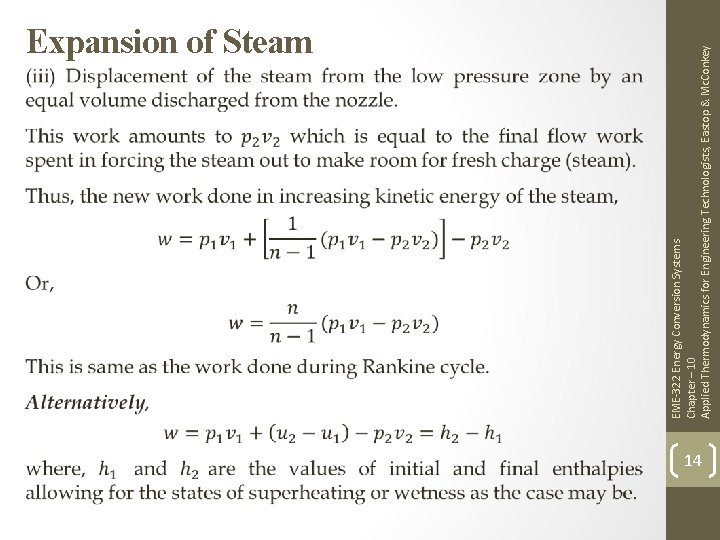
EME-322 Energy Conversion Systems Chapter – 10 Applied Thermodynamics for Engineering Technologists, Eastop & Mc. Conkey Expansion of Steam 14
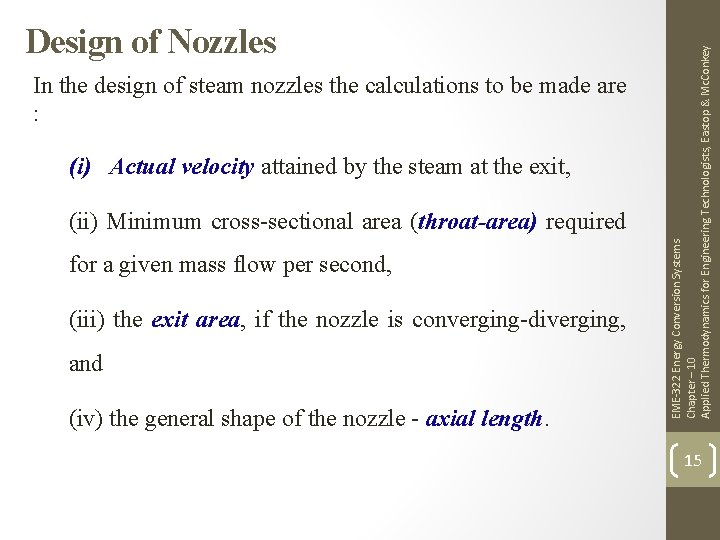
In the design of steam nozzles the calculations to be made are : (i) Actual velocity attained by the steam at the exit, (ii) Minimum cross-sectional area (throat-area) required for a given mass flow per second, (iii) the exit area, if the nozzle is converging-diverging, and (iv) the general shape of the nozzle - axial length. EME-322 Energy Conversion Systems Chapter – 10 Applied Thermodynamics for Engineering Technologists, Eastop & Mc. Conkey Design of Nozzles 15
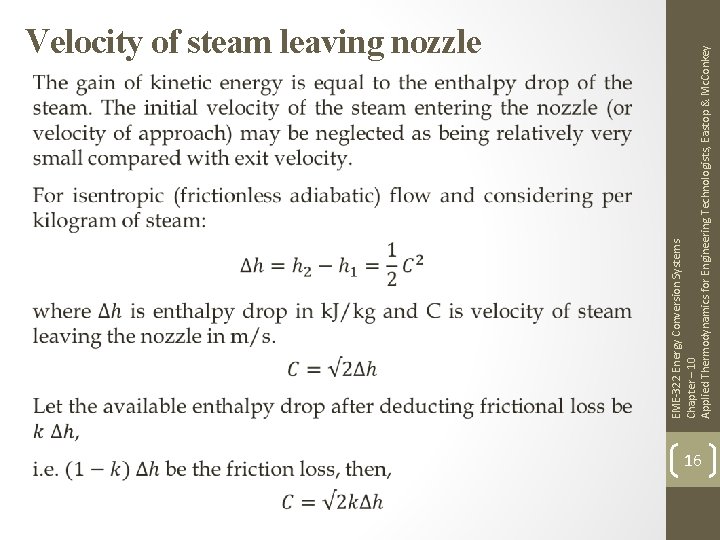
EME-322 Energy Conversion Systems Chapter – 10 Applied Thermodynamics for Engineering Technologists, Eastop & Mc. Conkey Velocity of steam leaving nozzle 16
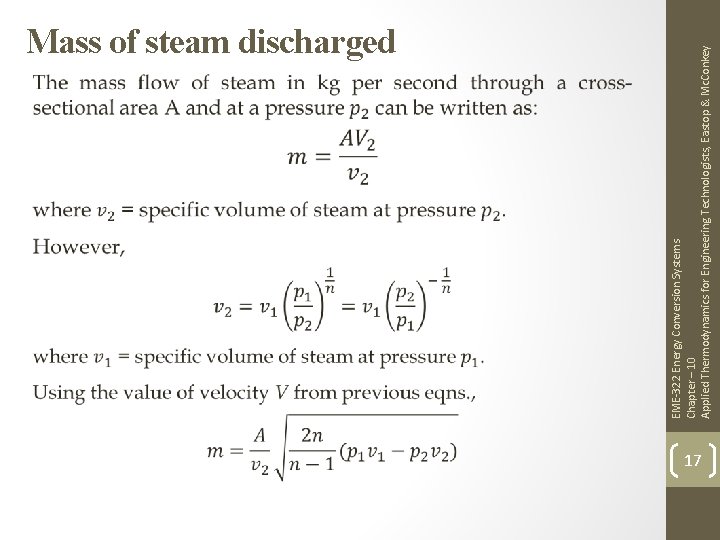
EME-322 Energy Conversion Systems Chapter – 10 Applied Thermodynamics for Engineering Technologists, Eastop & Mc. Conkey Mass of steam discharged 17
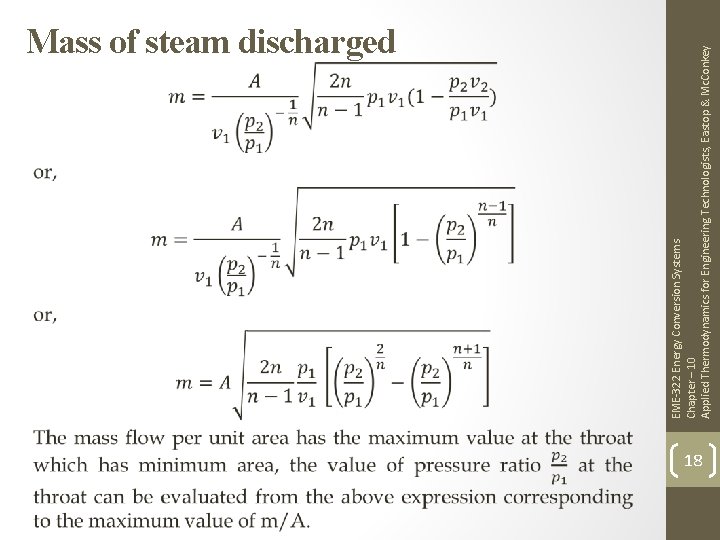
EME-322 Energy Conversion Systems Chapter – 10 Applied Thermodynamics for Engineering Technologists, Eastop & Mc. Conkey Mass of steam discharged 18
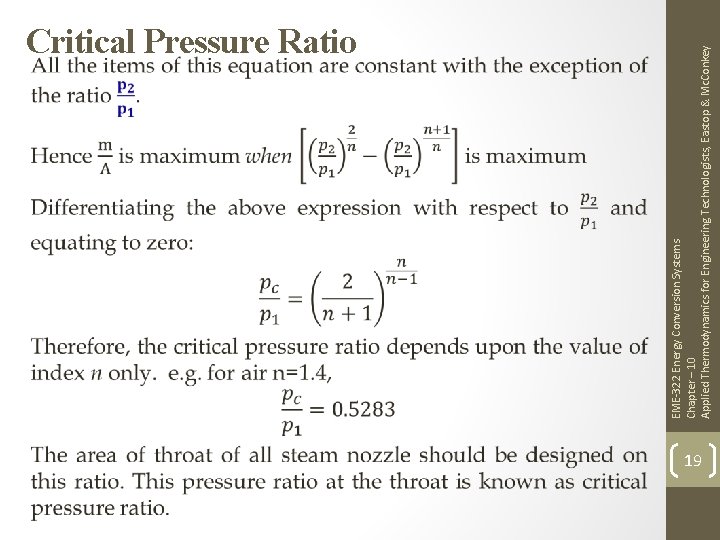
EME-322 Energy Conversion Systems Chapter – 10 Applied Thermodynamics for Engineering Technologists, Eastop & Mc. Conkey Critical Pressure Ratio 19
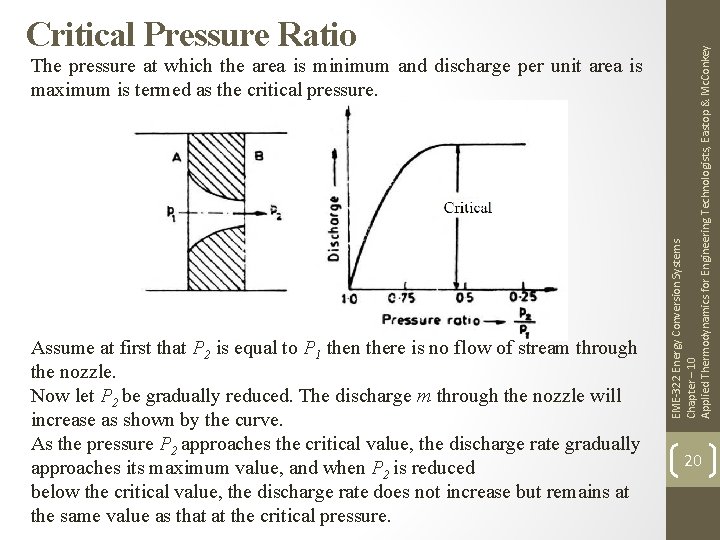
The pressure at which the area is minimum and discharge per unit area is maximum is termed as the critical pressure. Assume at first that P 2 is equal to P 1 then there is no flow of stream through the nozzle. Now let P 2 be gradually reduced. The discharge m through the nozzle will increase as shown by the curve. As the pressure P 2 approaches the critical value, the discharge rate gradually approaches its maximum value, and when P 2 is reduced below the critical value, the discharge rate does not increase but remains at the same value as that at the critical pressure. EME-322 Energy Conversion Systems Chapter – 10 Applied Thermodynamics for Engineering Technologists, Eastop & Mc. Conkey Critical Pressure Ratio 20
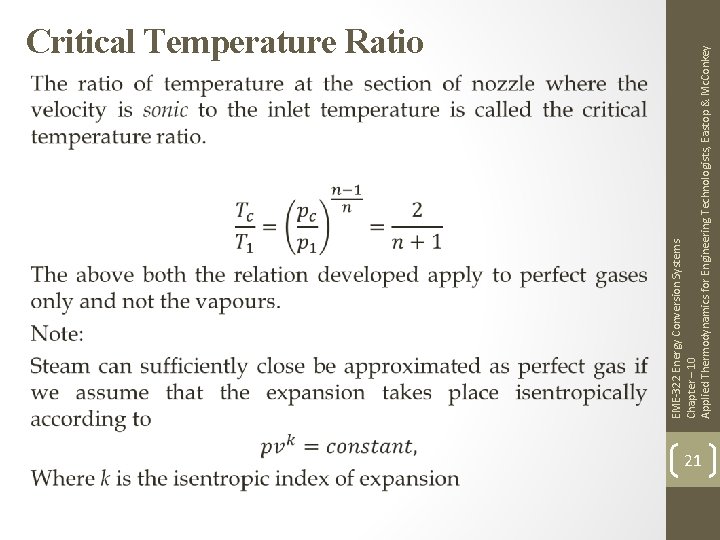
EME-322 Energy Conversion Systems Chapter – 10 Applied Thermodynamics for Engineering Technologists, Eastop & Mc. Conkey Critical Temperature Ratio 21
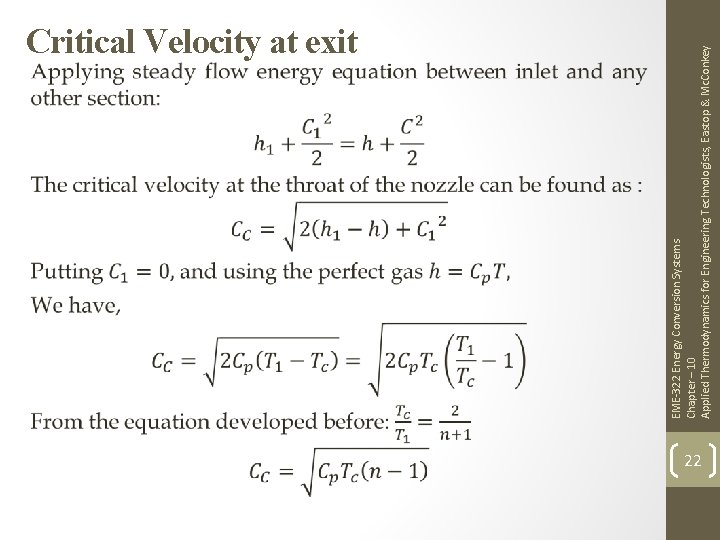
EME-322 Energy Conversion Systems Chapter – 10 Applied Thermodynamics for Engineering Technologists, Eastop & Mc. Conkey Critical Velocity at exit 22
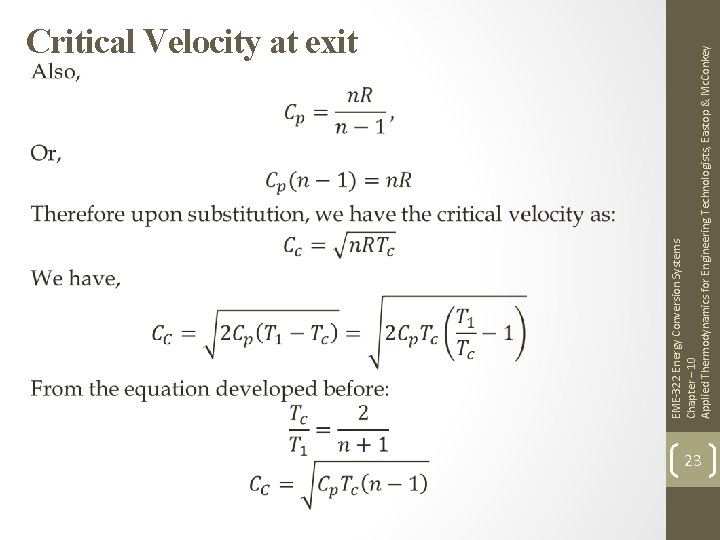
EME-322 Energy Conversion Systems Chapter – 10 Applied Thermodynamics for Engineering Technologists, Eastop & Mc. Conkey Critical Velocity at exit 23
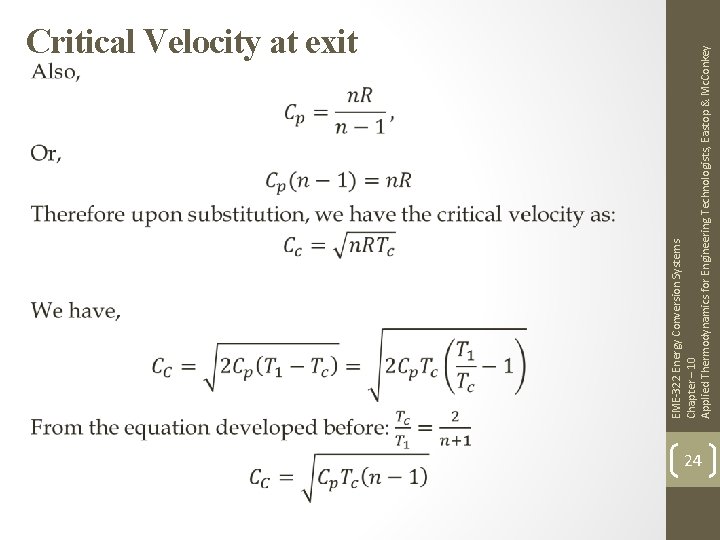
EME-322 Energy Conversion Systems Chapter – 10 Applied Thermodynamics for Engineering Technologists, Eastop & Mc. Conkey Critical Velocity at exit 24
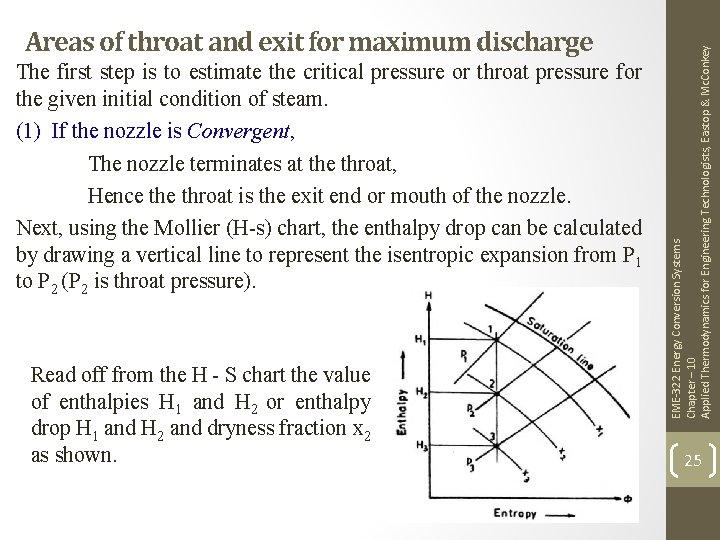
The first step is to estimate the critical pressure or throat pressure for the given initial condition of steam. (1) If the nozzle is Convergent, The nozzle terminates at the throat, Hence throat is the exit end or mouth of the nozzle. Next, using the Mollier (H-s) chart, the enthalpy drop can be calculated by drawing a vertical line to represent the isentropic expansion from P 1 to P 2 (P 2 is throat pressure). Read off from the H - S chart the value of enthalpies H 1 and H 2 or enthalpy drop H 1 and H 2 and dryness fraction x 2 as shown. EME-322 Energy Conversion Systems Chapter – 10 Applied Thermodynamics for Engineering Technologists, Eastop & Mc. Conkey Areas of throat and exit for maximum discharge 25
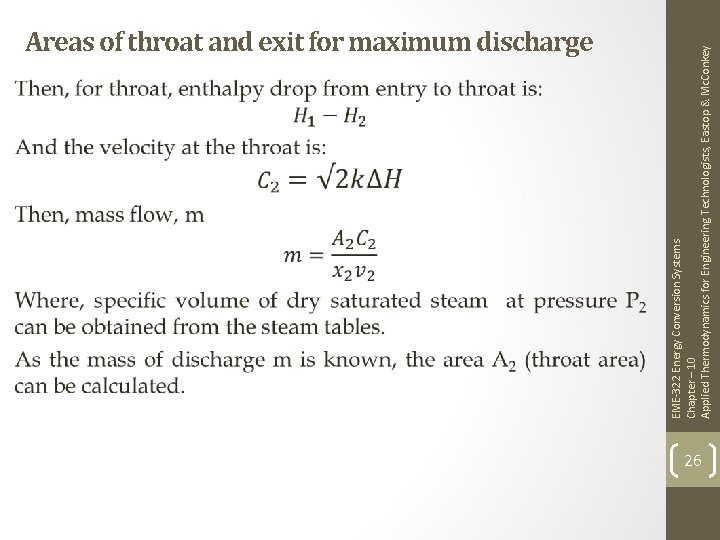
EME-322 Energy Conversion Systems Chapter – 10 Applied Thermodynamics for Engineering Technologists, Eastop & Mc. Conkey Areas of throat and exit for maximum discharge 26
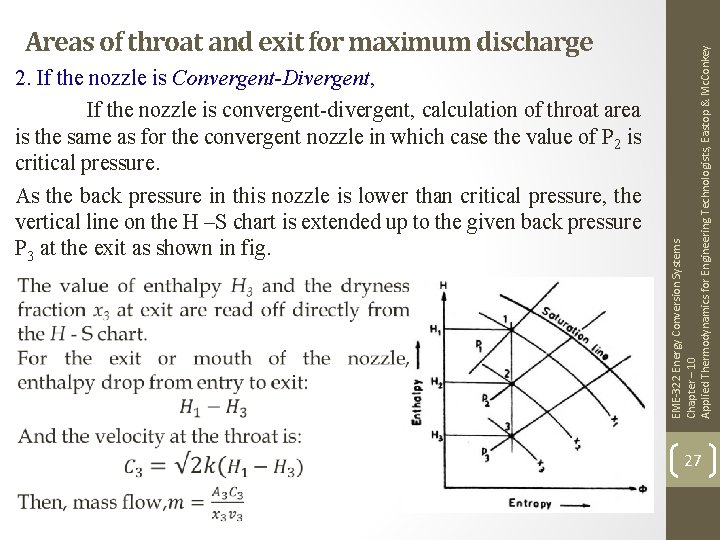
2. If the nozzle is Convergent-Divergent, If the nozzle is convergent-divergent, calculation of throat area is the same as for the convergent nozzle in which case the value of P 2 is critical pressure. As the back pressure in this nozzle is lower than critical pressure, the vertical line on the H –S chart is extended up to the given back pressure P 3 at the exit as shown in fig. EME-322 Energy Conversion Systems Chapter – 10 Applied Thermodynamics for Engineering Technologists, Eastop & Mc. Conkey Areas of throat and exit for maximum discharge 27
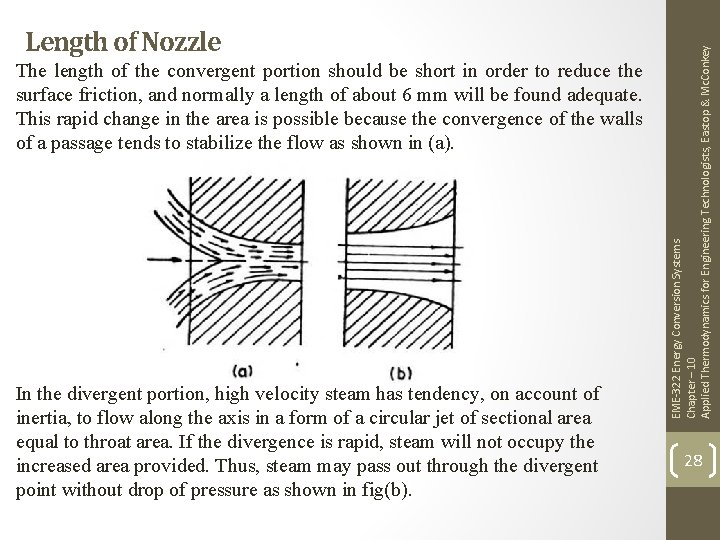
The length of the convergent portion should be short in order to reduce the surface friction, and normally a length of about 6 mm will be found adequate. This rapid change in the area is possible because the convergence of the walls of a passage tends to stabilize the flow as shown in (a). In the divergent portion, high velocity steam has tendency, on account of inertia, to flow along the axis in a form of a circular jet of sectional area equal to throat area. If the divergence is rapid, steam will not occupy the increased area provided. Thus, steam may pass out through the divergent point without drop of pressure as shown in fig(b). EME-322 Energy Conversion Systems Chapter – 10 Applied Thermodynamics for Engineering Technologists, Eastop & Mc. Conkey Length of Nozzle 28
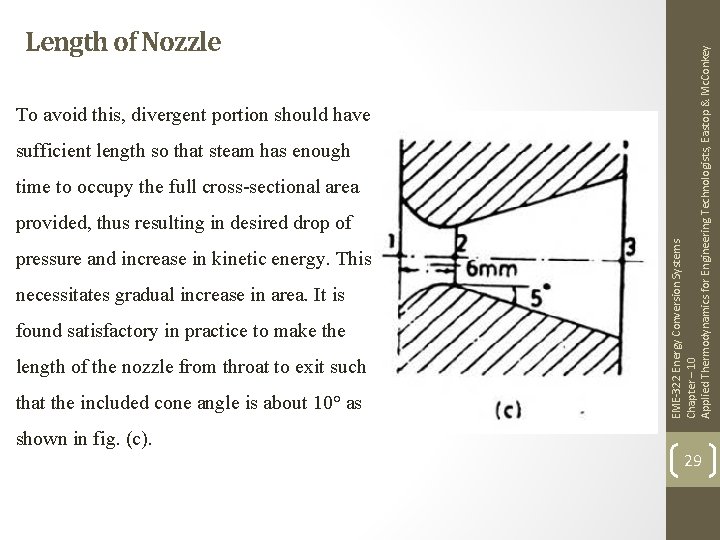
To avoid this, divergent portion should have sufficient length so that steam has enough time to occupy the full cross-sectional area provided, thus resulting in desired drop of pressure and increase in kinetic energy. This necessitates gradual increase in area. It is found satisfactory in practice to make the length of the nozzle from throat to exit such that the included cone angle is about 10° as shown in fig. (c). EME-322 Energy Conversion Systems Chapter – 10 Applied Thermodynamics for Engineering Technologists, Eastop & Mc. Conkey Length of Nozzle 29
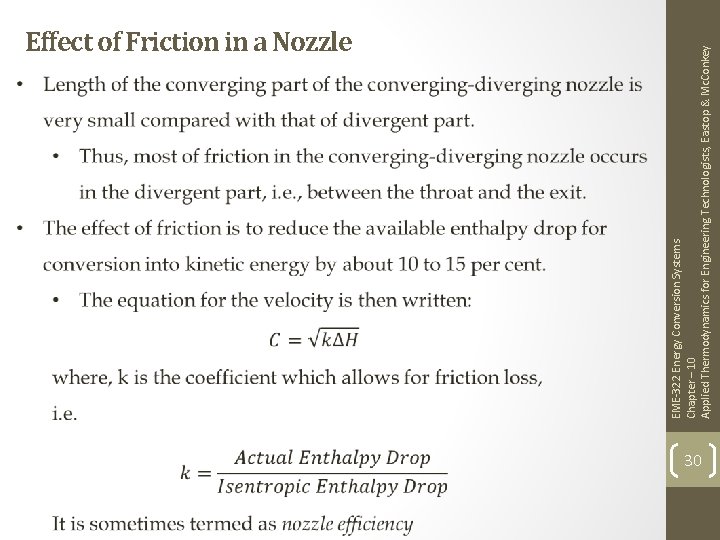
EME-322 Energy Conversion Systems Chapter – 10 Applied Thermodynamics for Engineering Technologists, Eastop & Mc. Conkey Effect of Friction in a Nozzle 30
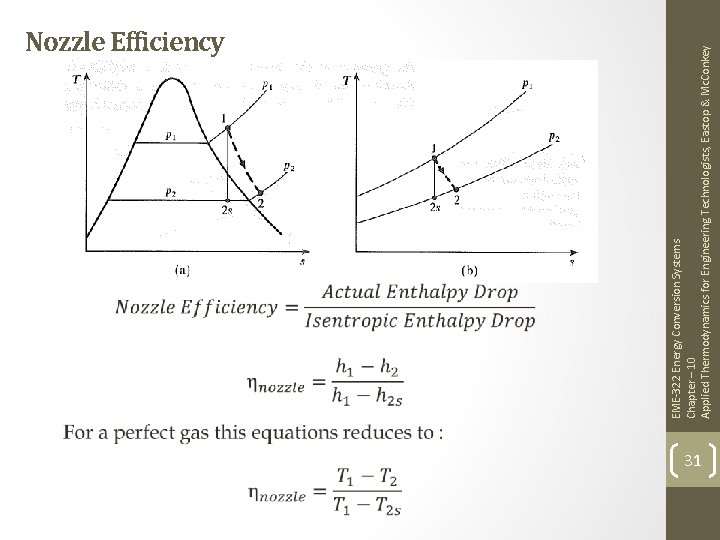
EME-322 Energy Conversion Systems Chapter – 10 Applied Thermodynamics for Engineering Technologists, Eastop & Mc. Conkey Nozzle Efficiency 31
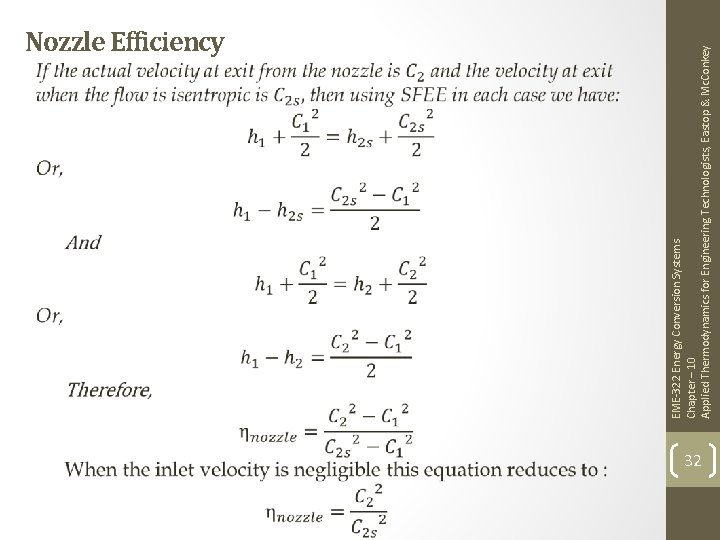
EME-322 Energy Conversion Systems Chapter – 10 Applied Thermodynamics for Engineering Technologists, Eastop & Mc. Conkey Nozzle Efficiency 32
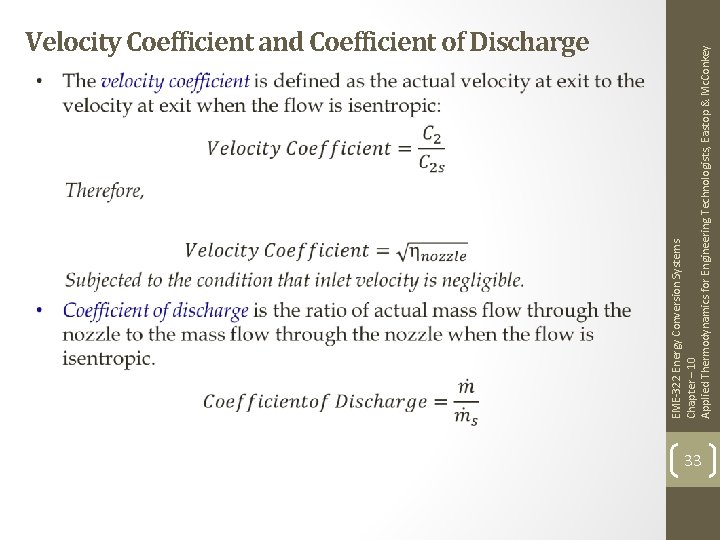
EME-322 Energy Conversion Systems Chapter – 10 Applied Thermodynamics for Engineering Technologists, Eastop & Mc. Conkey Velocity Coefficient and Coefficient of Discharge 33
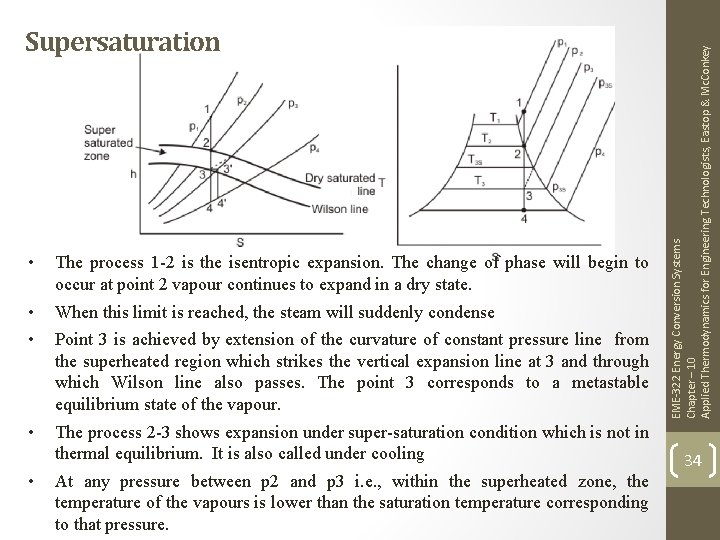
• The process 1 -2 is the isentropic expansion. The change of phase will begin to occur at point 2 vapour continues to expand in a dry state. • • When this limit is reached, the steam will suddenly condense • The process 2 -3 shows expansion under super-saturation condition which is not in thermal equilibrium. It is also called under cooling • Point 3 is achieved by extension of the curvature of constant pressure line from the superheated region which strikes the vertical expansion line at 3 and through which Wilson line also passes. The point 3 corresponds to a metastable equilibrium state of the vapour. At any pressure between p 2 and p 3 i. e. , within the superheated zone, the temperature of the vapours is lower than the saturation temperature corresponding to that pressure. EME-322 Energy Conversion Systems Chapter – 10 Applied Thermodynamics for Engineering Technologists, Eastop & Mc. Conkey Supersaturation 34
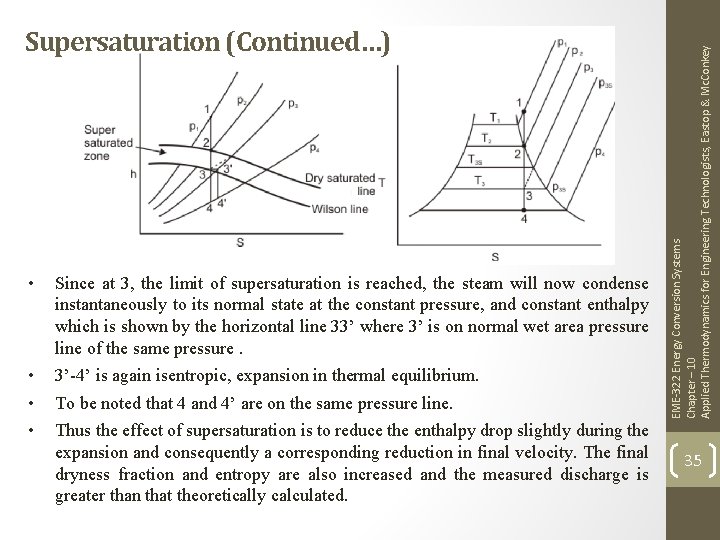
• Since at 3, the limit of supersaturation is reached, the steam will now condense instantaneously to its normal state at the constant pressure, and constant enthalpy which is shown by the horizontal line 33’ where 3’ is on normal wet area pressure line of the same pressure. • • • 3’-4’ is again isentropic, expansion in thermal equilibrium. To be noted that 4 and 4’ are on the same pressure line. Thus the effect of supersaturation is to reduce the enthalpy drop slightly during the expansion and consequently a corresponding reduction in final velocity. The final dryness fraction and entropy are also increased and the measured discharge is greater than that theoretically calculated. EME-322 Energy Conversion Systems Chapter – 10 Applied Thermodynamics for Engineering Technologists, Eastop & Mc. Conkey Supersaturation (Continued…) 35
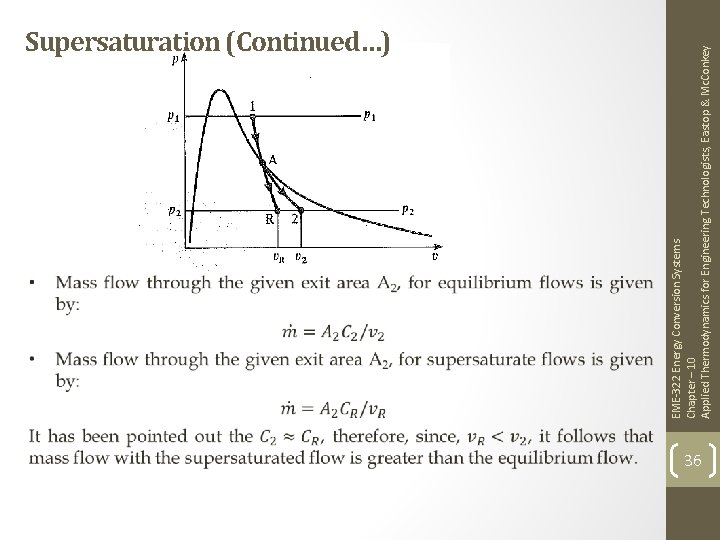
EME-322 Energy Conversion Systems Chapter – 10 Applied Thermodynamics for Engineering Technologists, Eastop & Mc. Conkey Supersaturation (Continued…) 36
Pharmacology and venipuncture in radiology pdf
Reschual
Chemical engineering thermodynamics 8th solution chapter 3
Thermodynamics for chemical engineering
Chemical engineering thermodynamics 8th solution chapter 6
Chemical engineering thermodynamics 8th solution chapter 10
熱力
Introduction to chemical engineering thermodynamics
Engineering thermodynamics
Feedwater
Thermodynamics an engineering approach
Thermodynamics an engineering approach
Applied geotechnical engineering
Principles of applied engineering
Fu foundation school of engineering
Uc college of engineering and applied science
Gerling applied engineering
Thermodynamics chapter 2
Thermodynamics chapter 4
What is cp in thermodynamics
Q=w/j
Formuö
Typiska novell drag
Tack för att ni lyssnade bild
Vad står k.r.å.k.a.n för
Varför kallas perioden 1918-1939 för mellankrigstiden
En lathund för arbete med kontinuitetshantering
Adressändring ideell förening
Tidbok för yrkesförare
Anatomi organ reproduksi
Förklara densitet för barn
Datorkunskap för nybörjare
Stig kerman
Mall för debattartikel
Magnetsjukhus
Nyckelkompetenser för livslångt lärande
Påbyggnader för flakfordon