Canadian Nuclear Society Ionising Radiation Workshop CNS Team
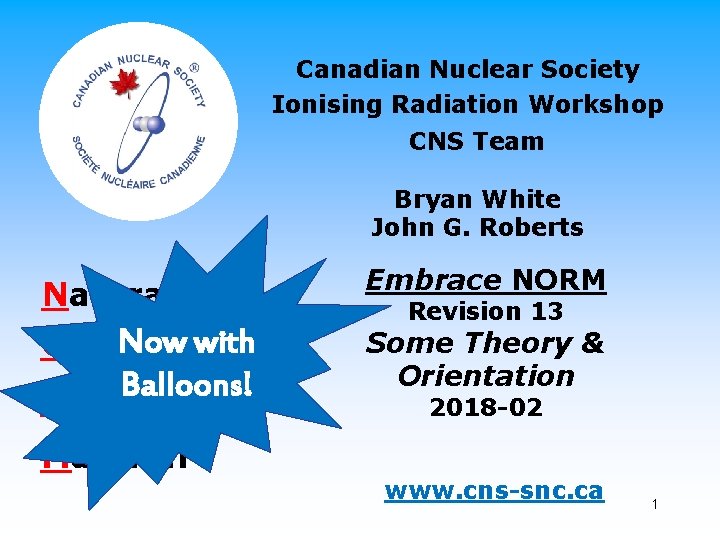
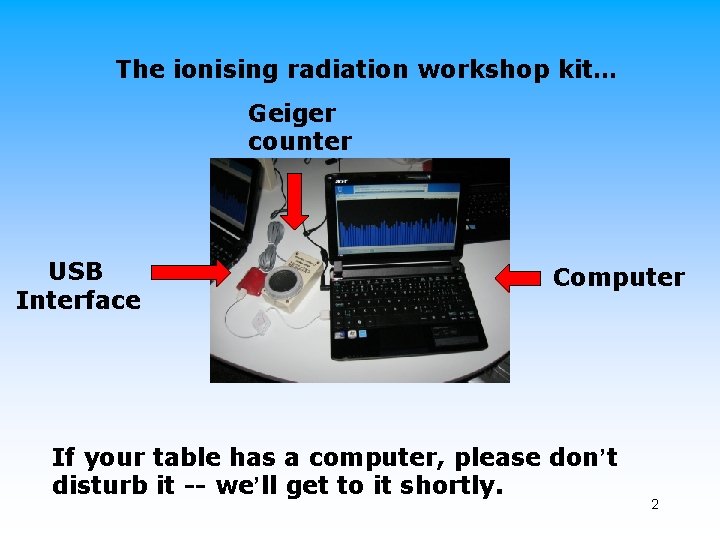
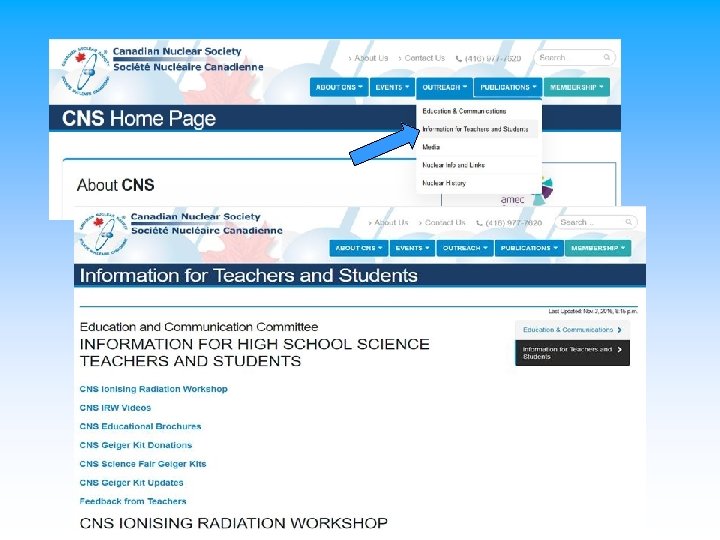
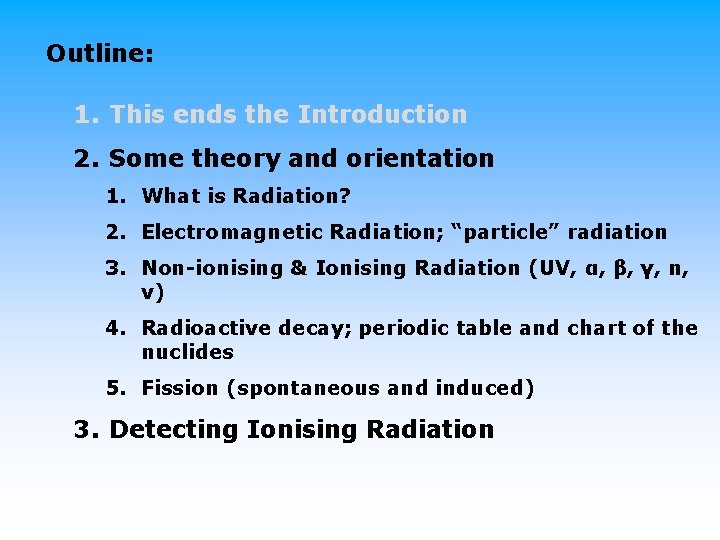
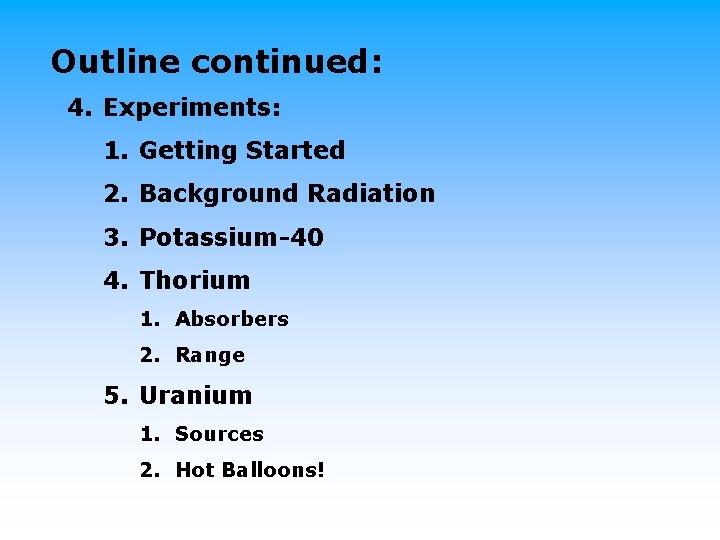
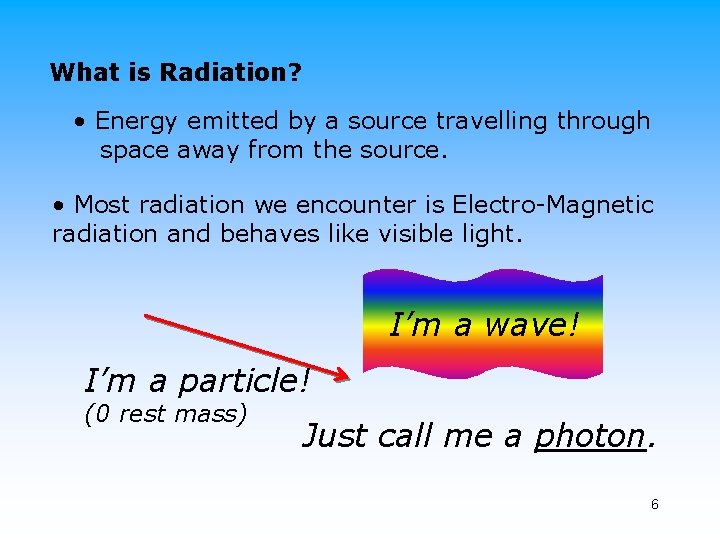
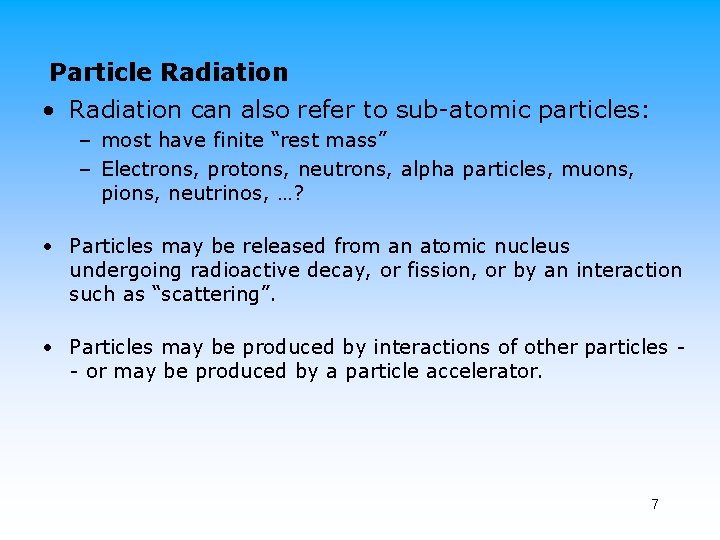
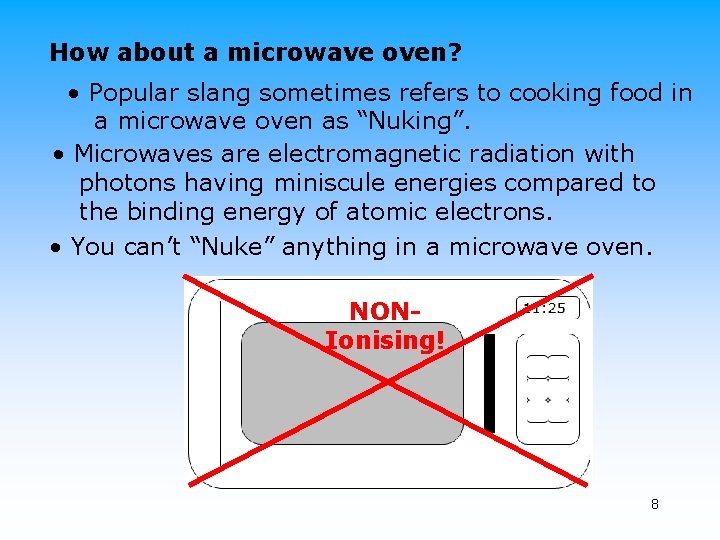
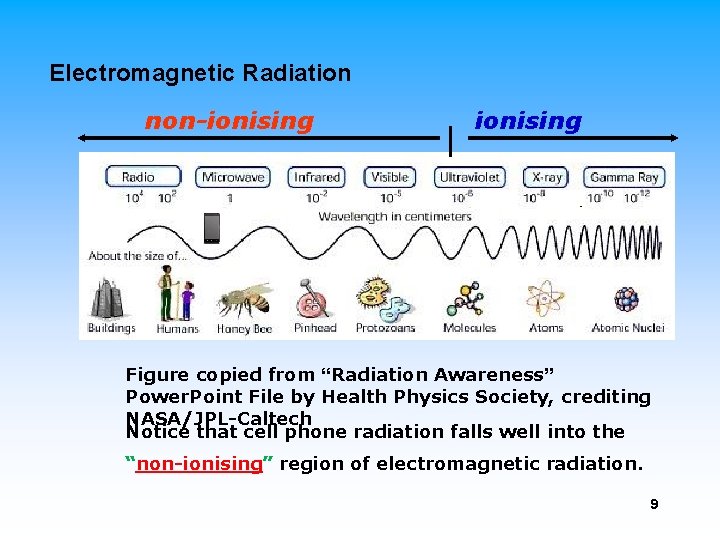
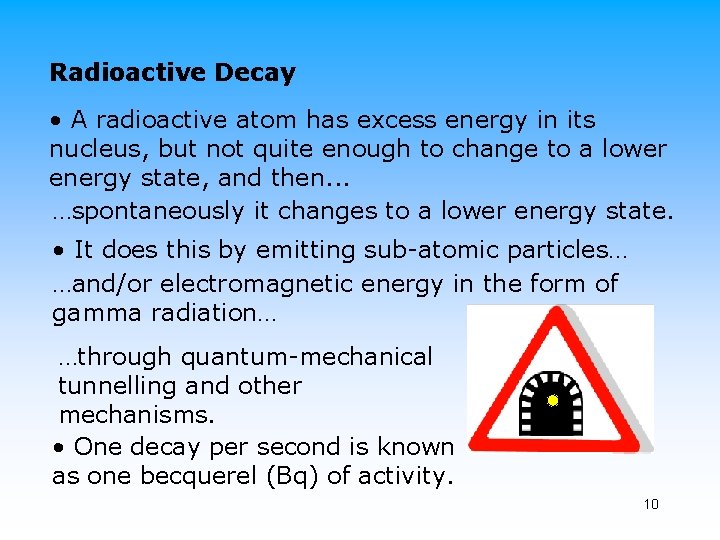
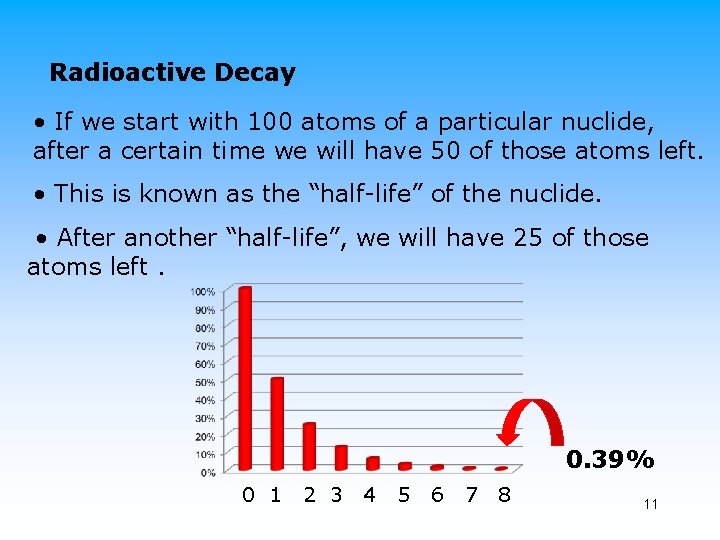
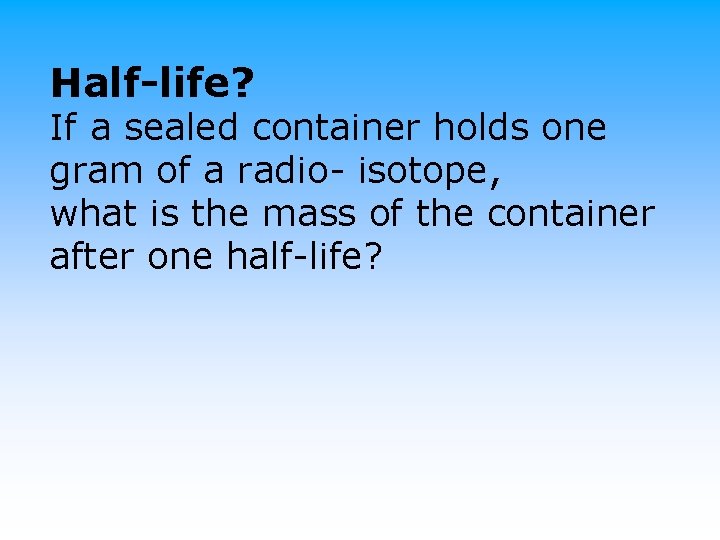
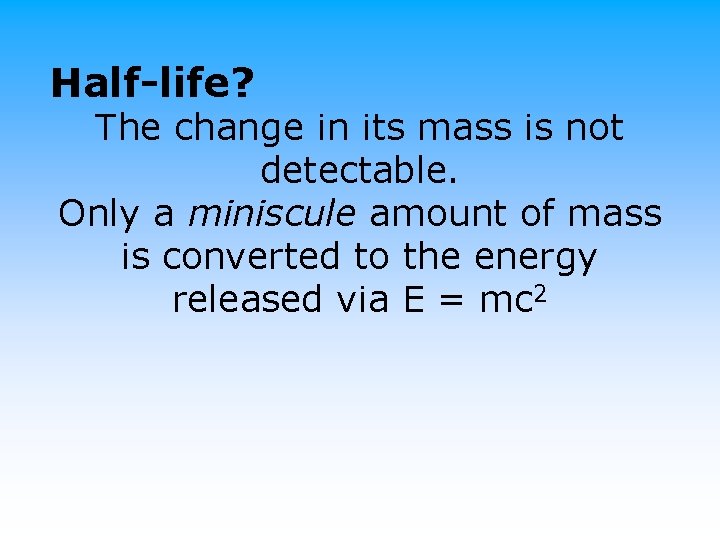
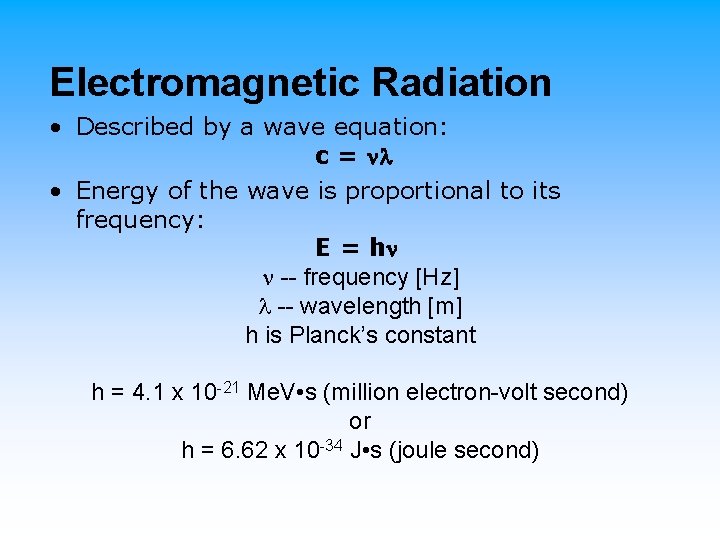
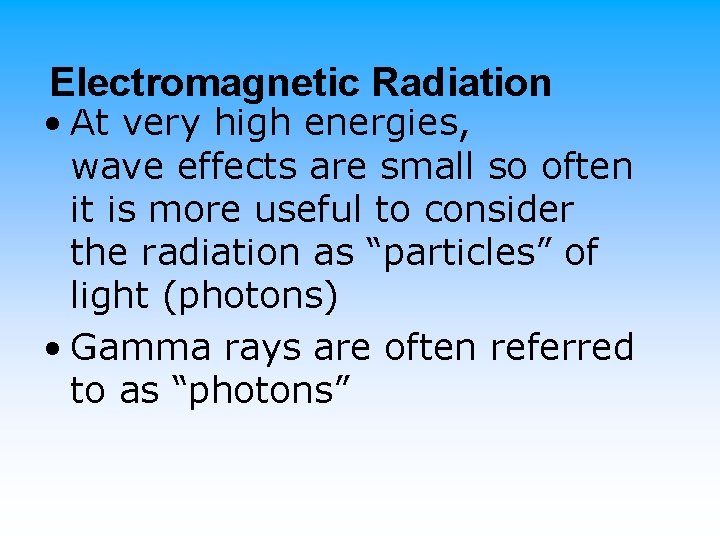
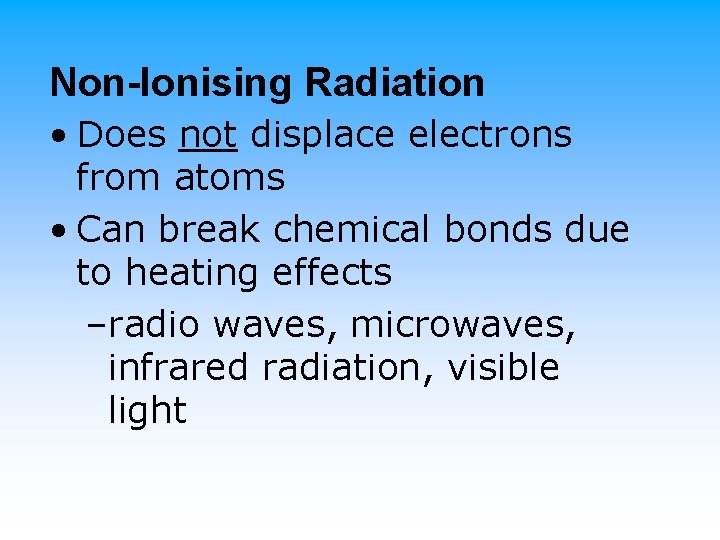
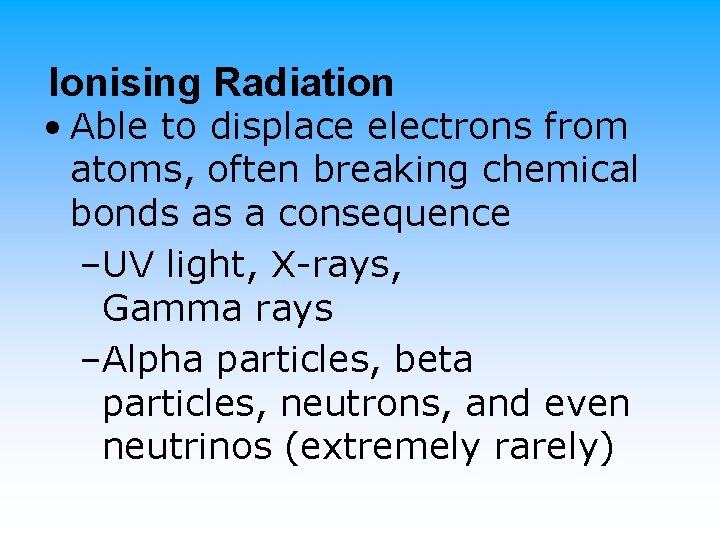
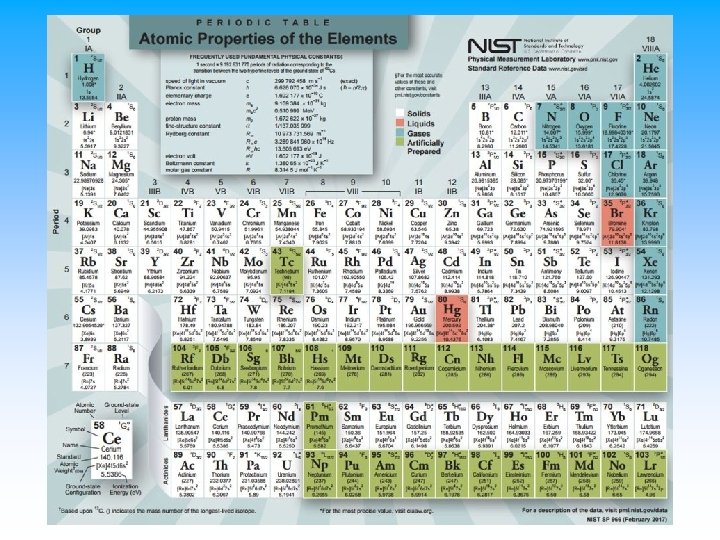
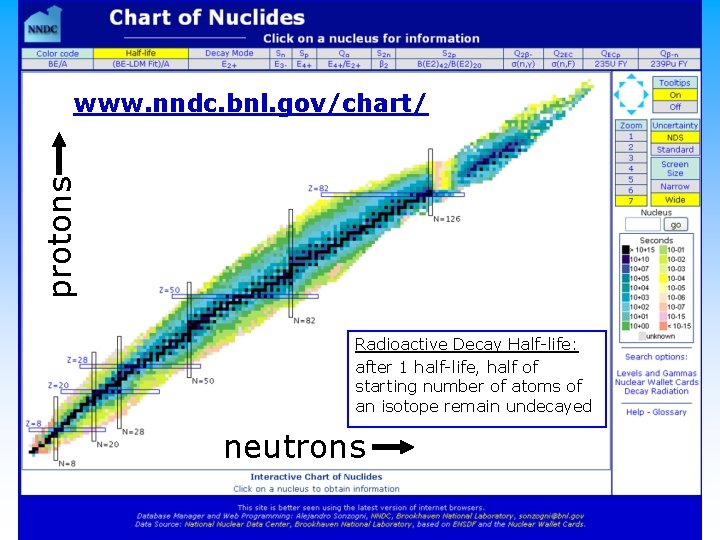
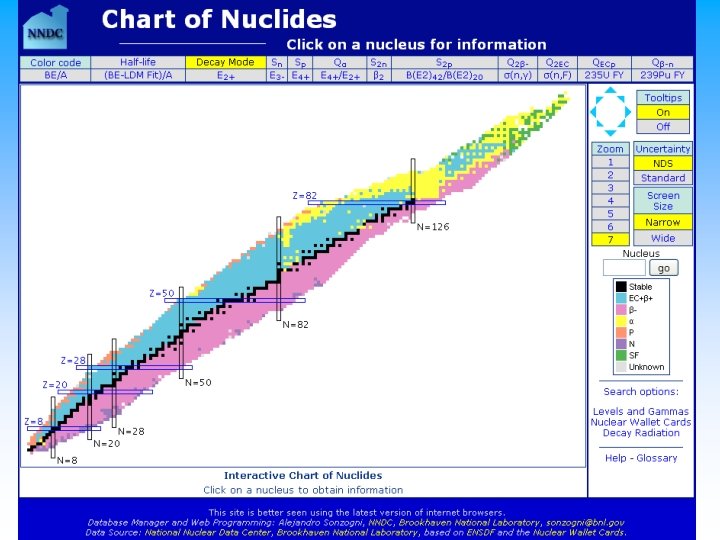
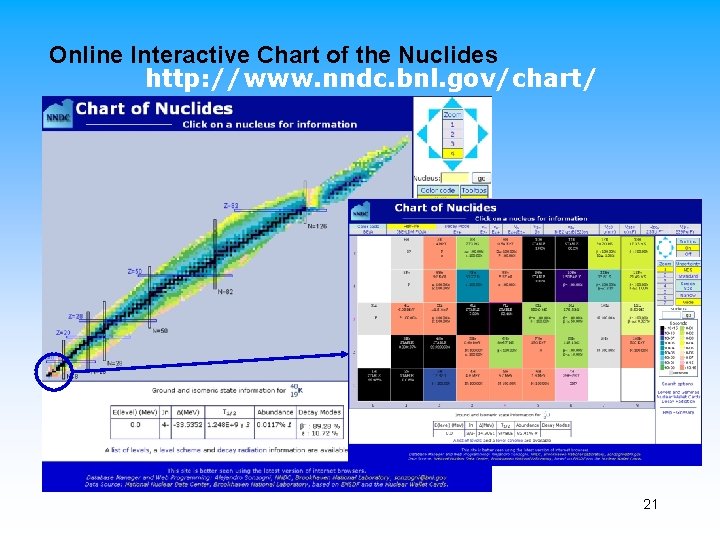
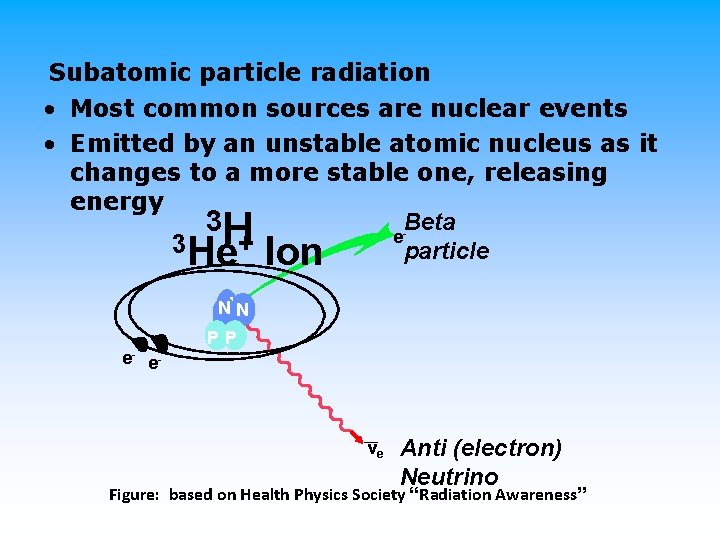
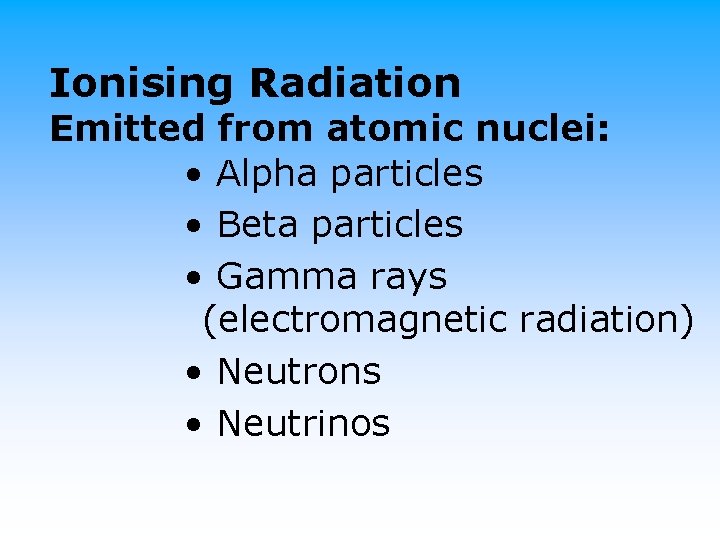
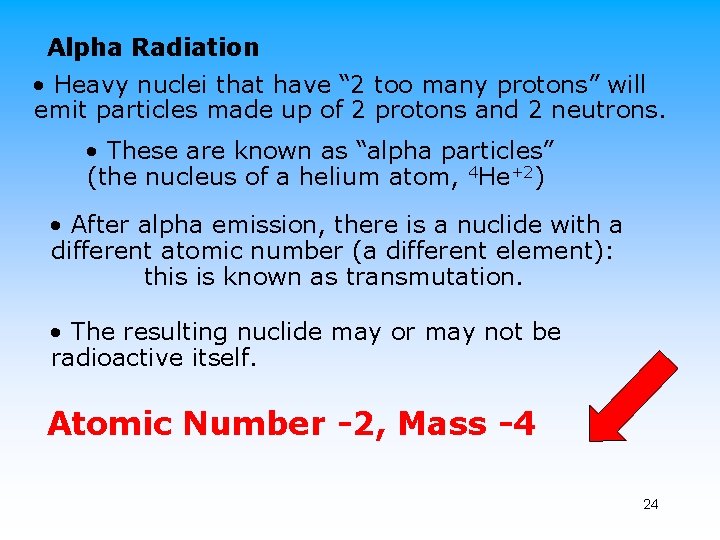
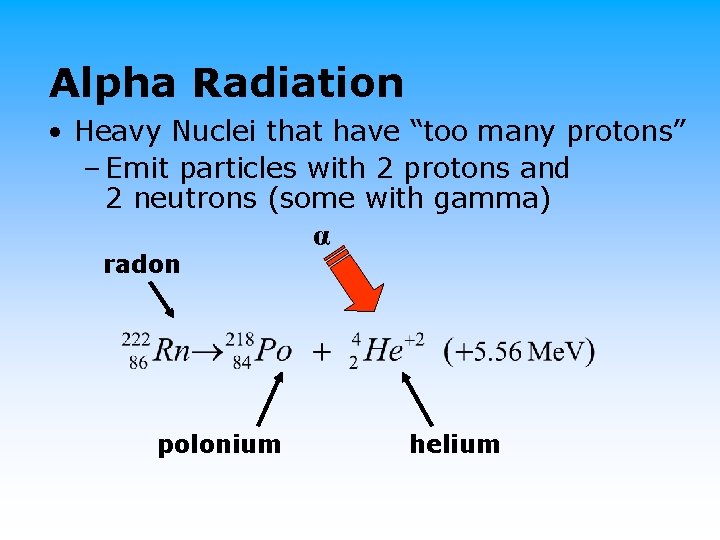
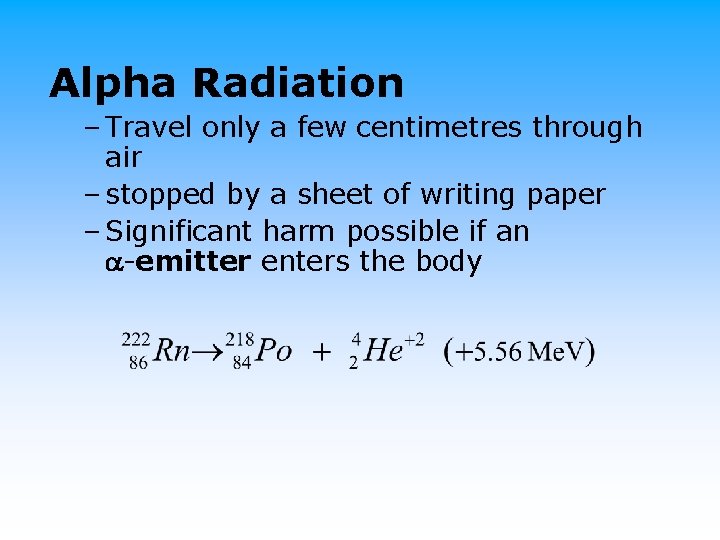
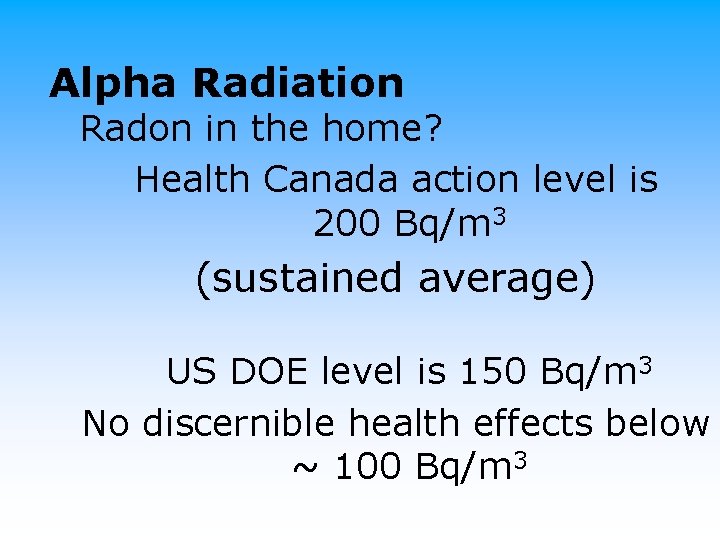
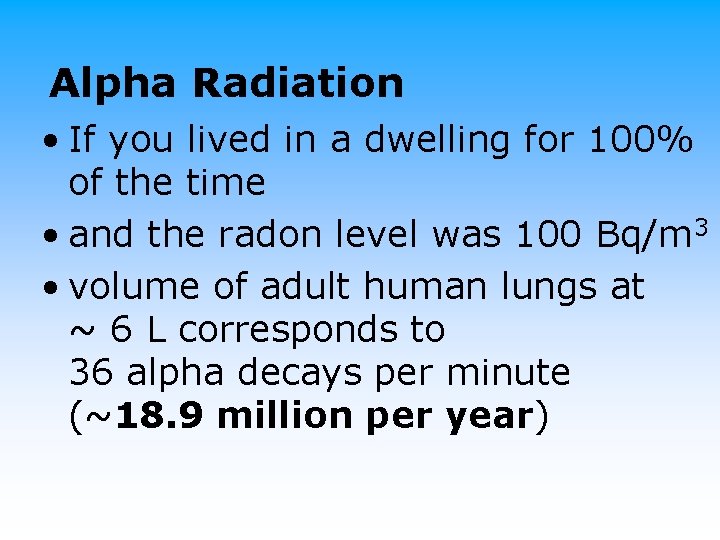
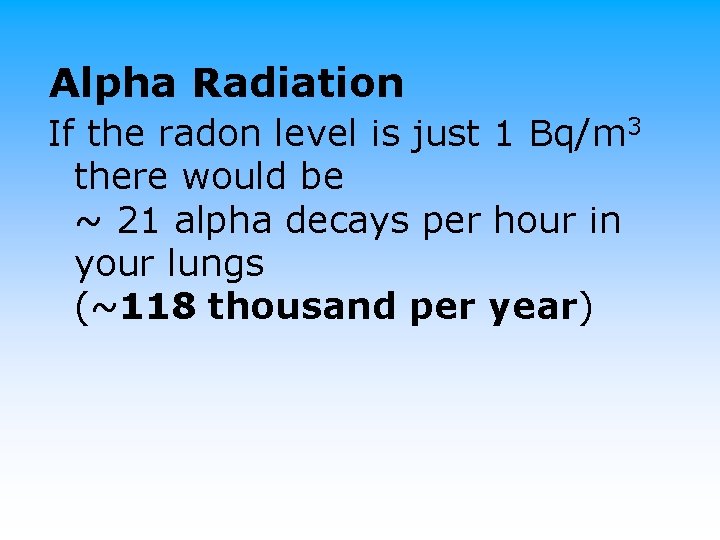
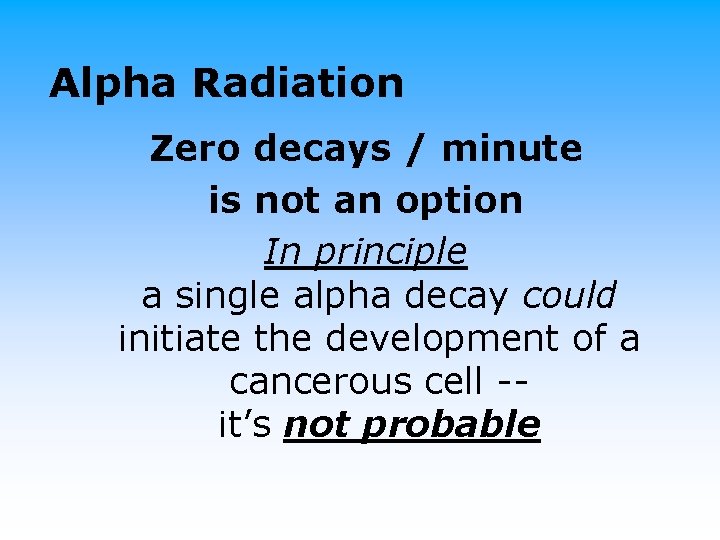
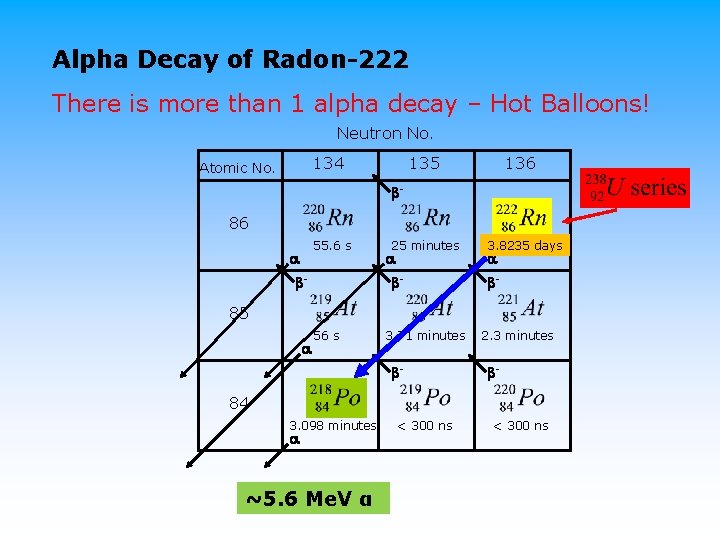
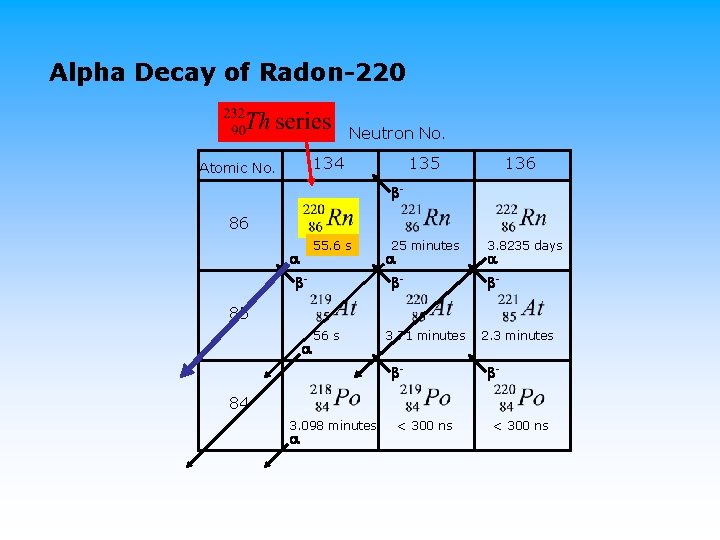
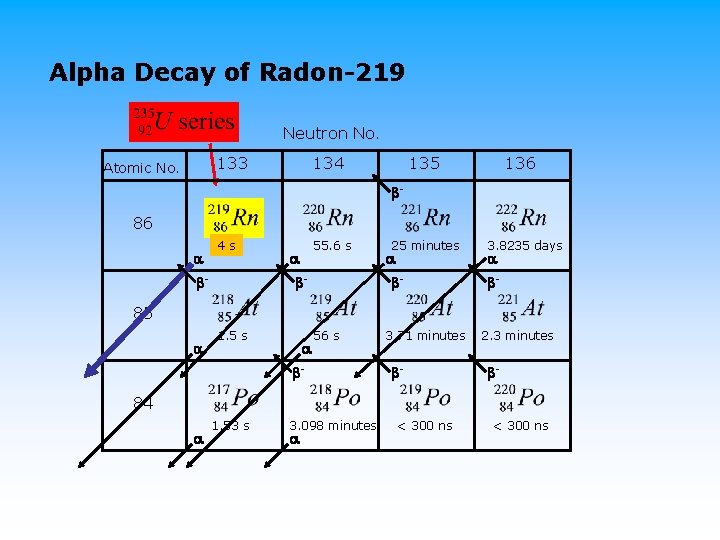
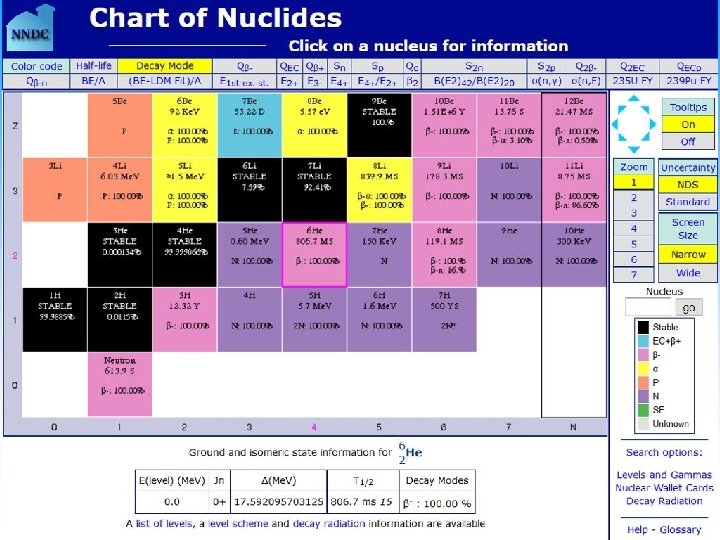
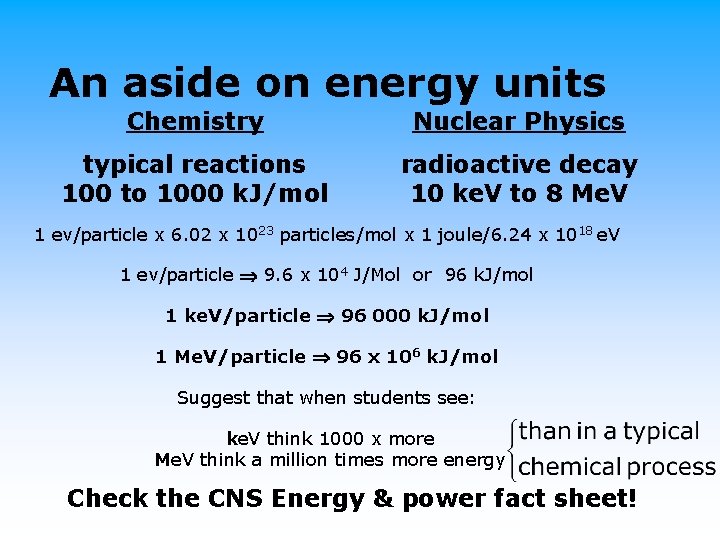
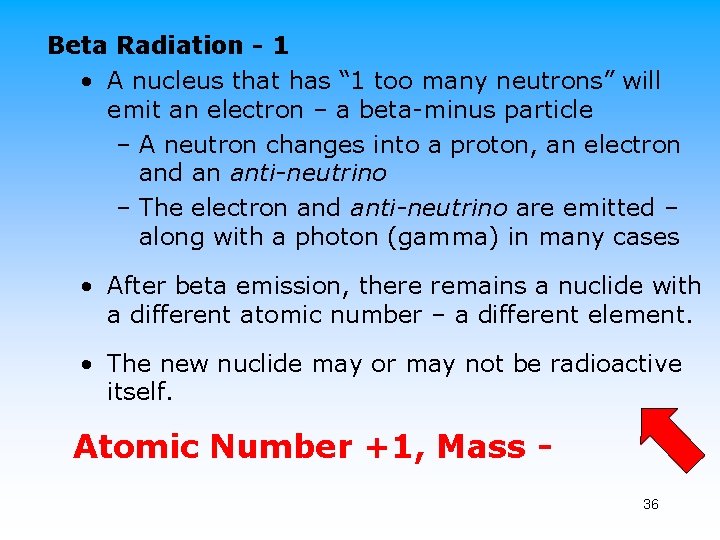
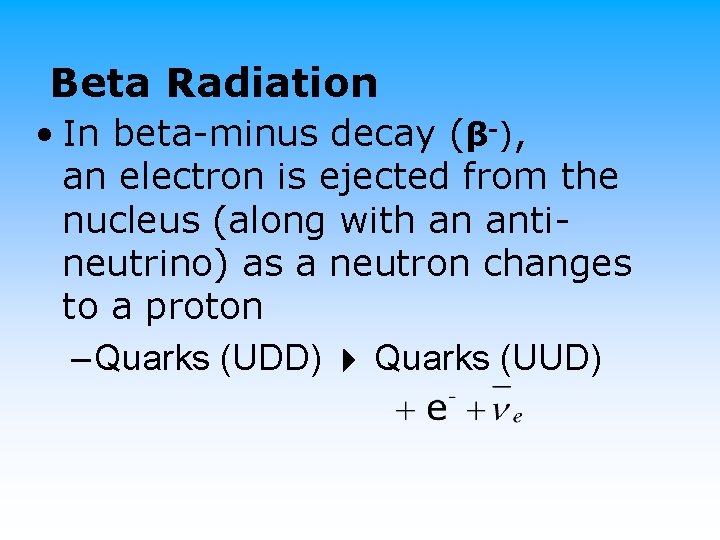
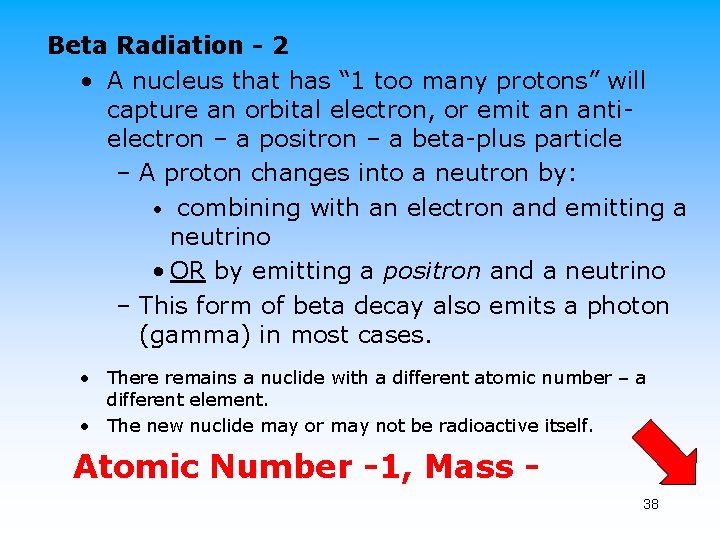
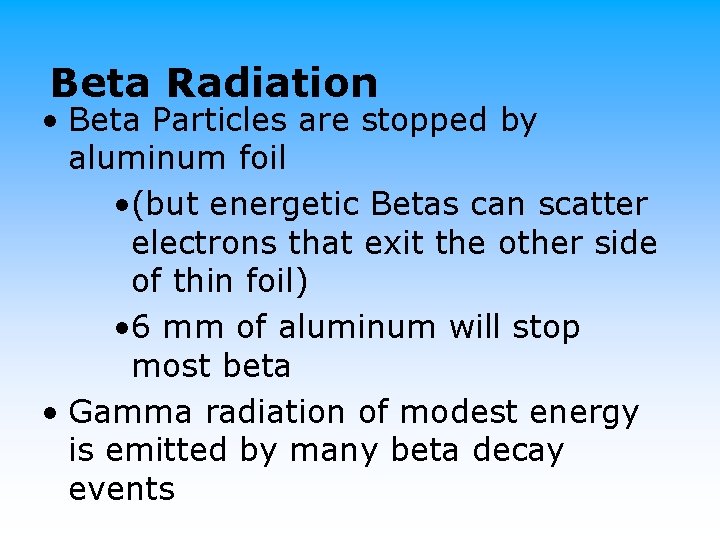
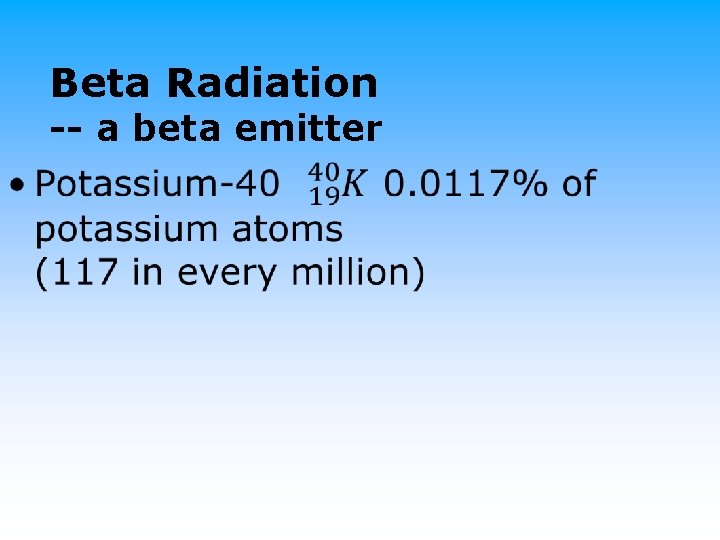
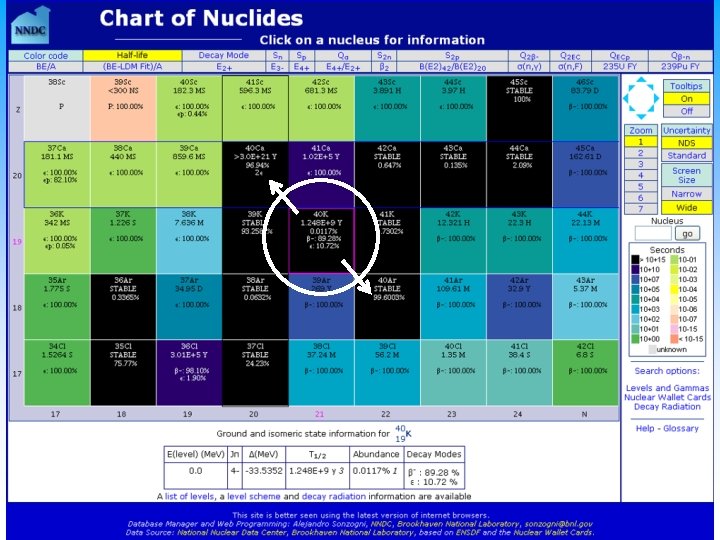
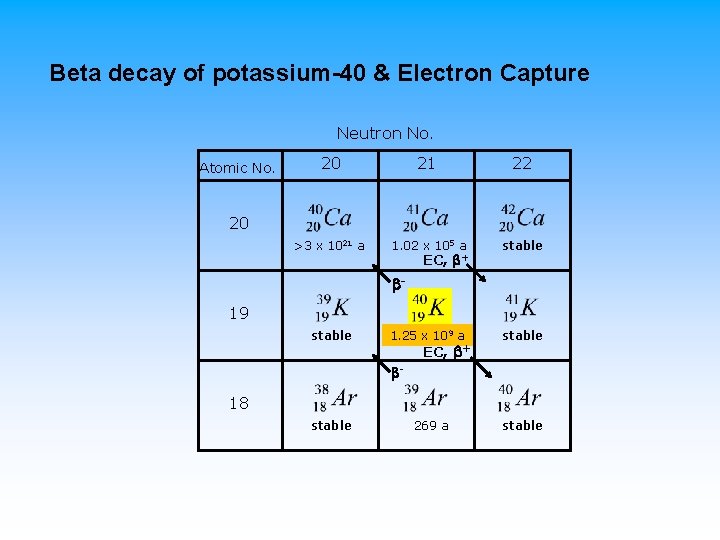
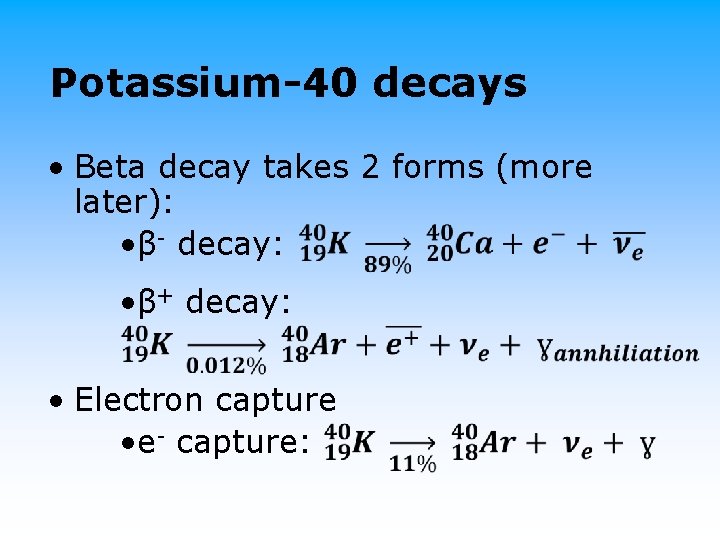
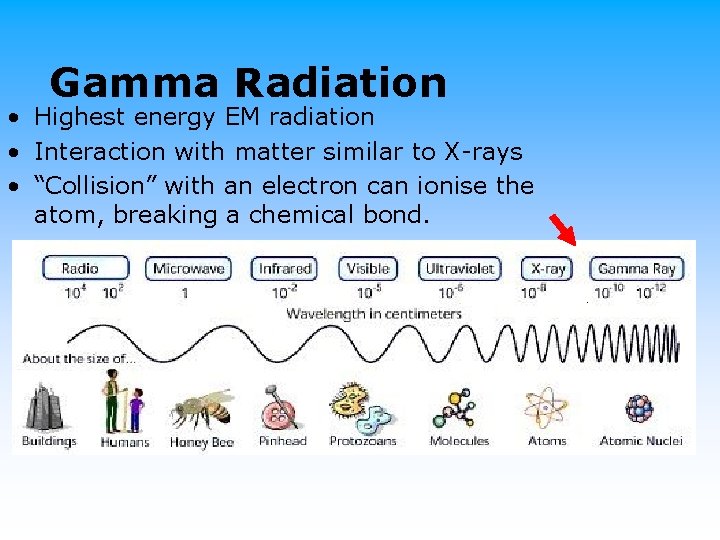
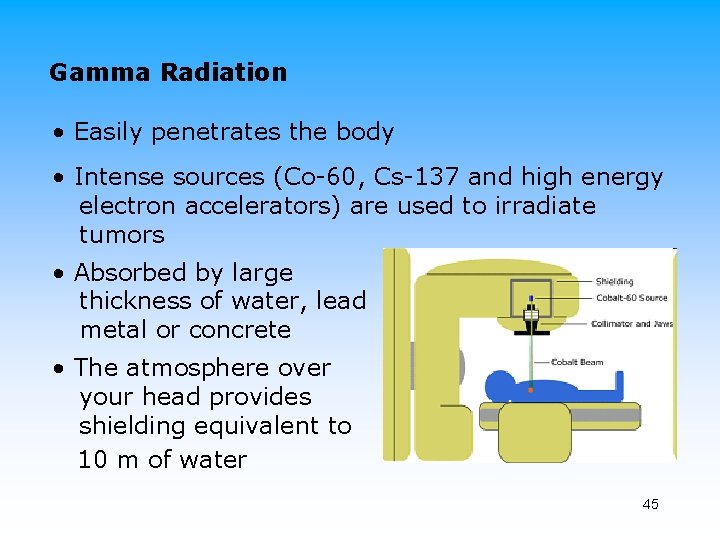
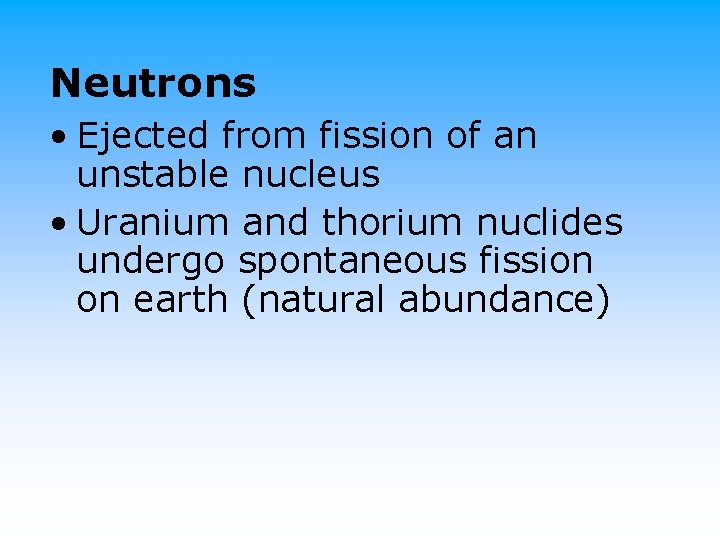
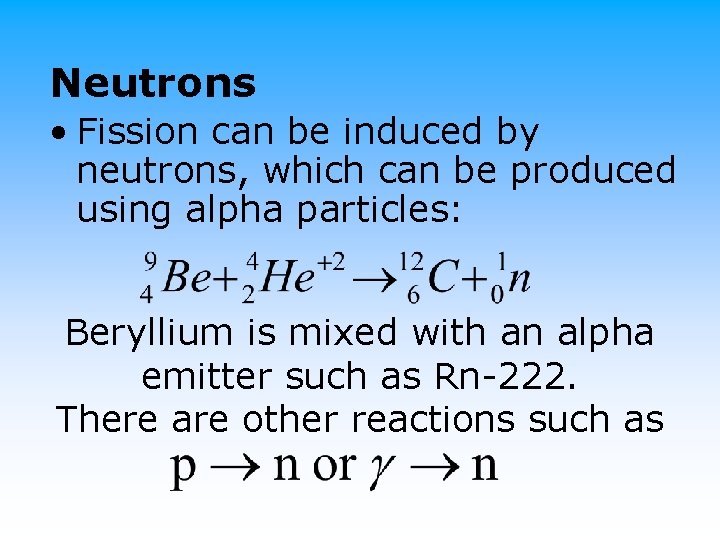
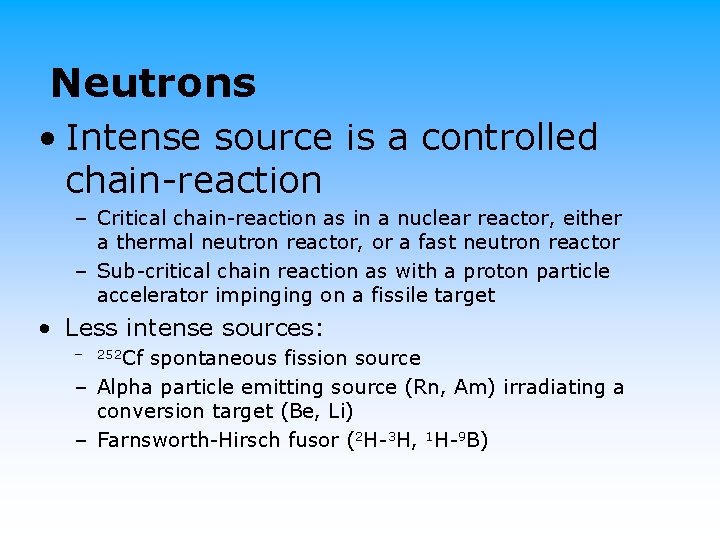
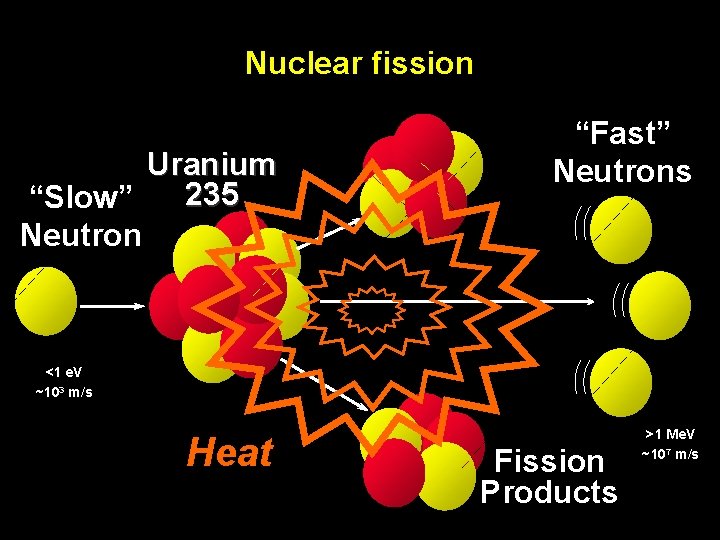
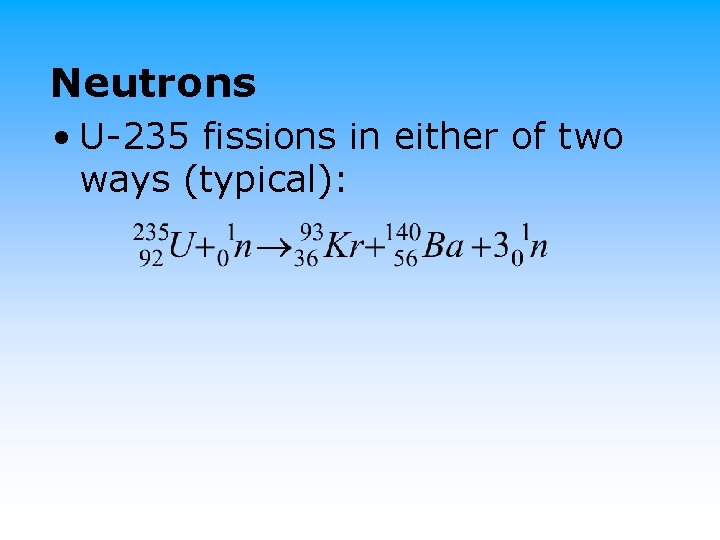
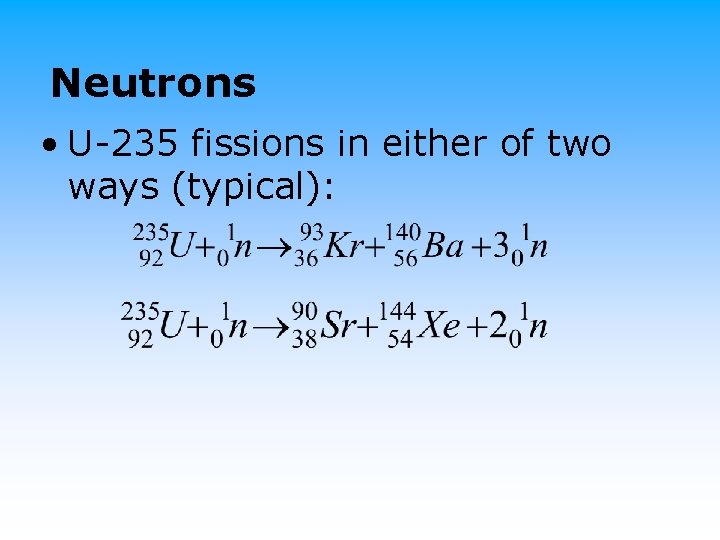
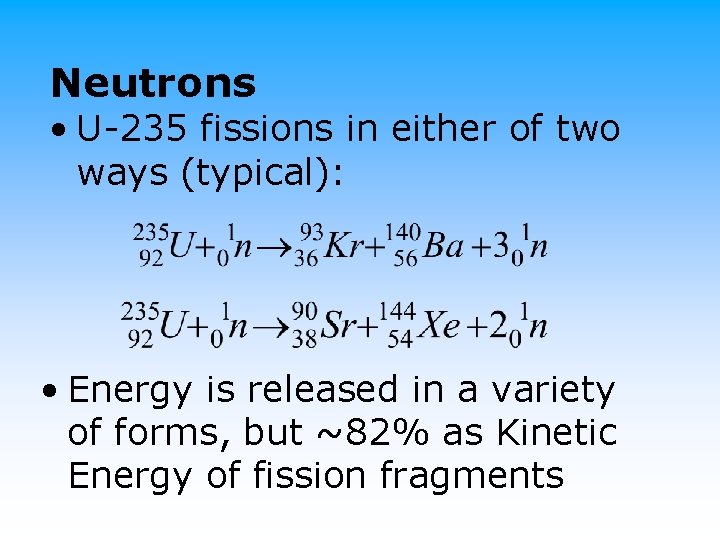
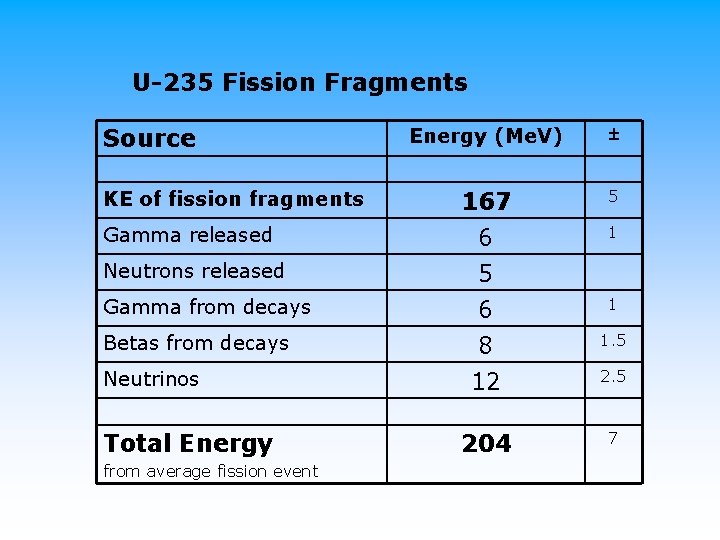
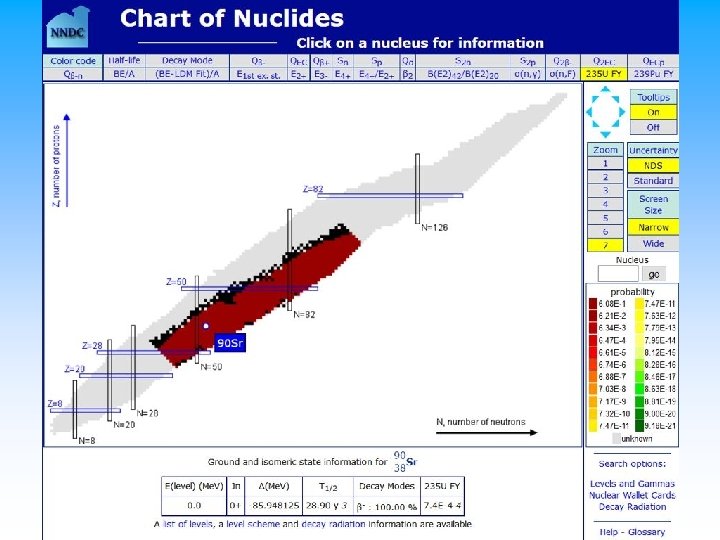
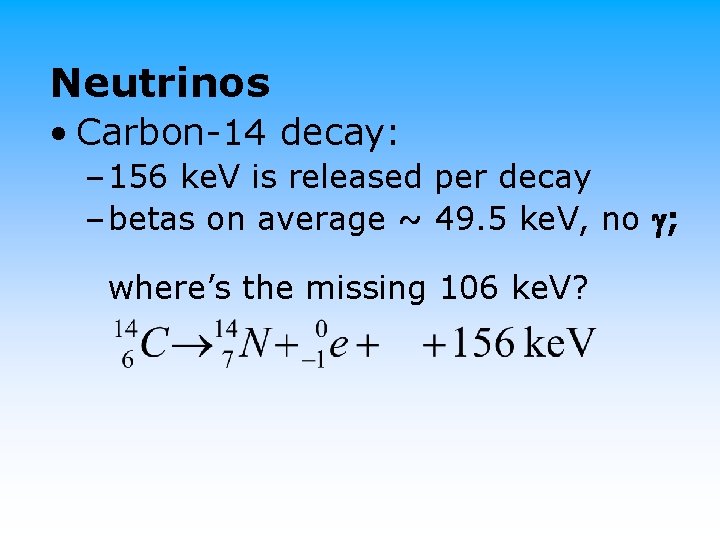
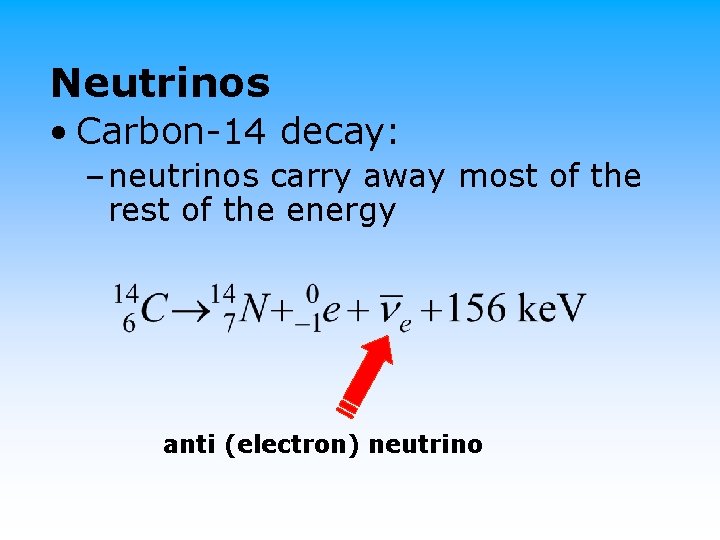
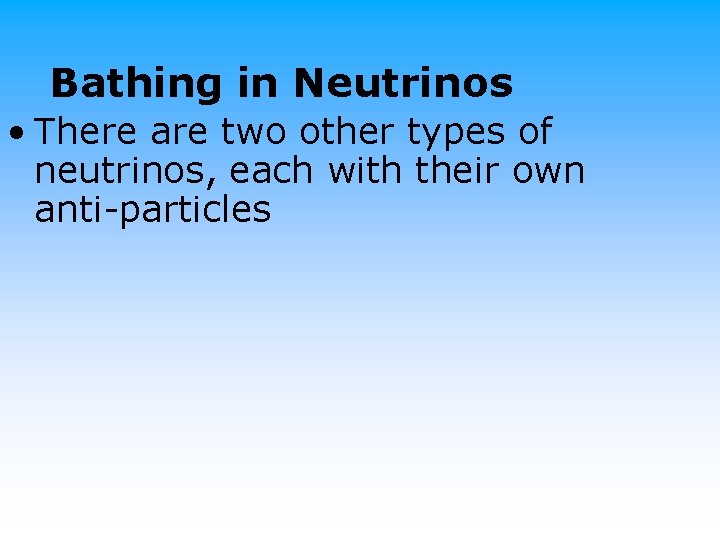
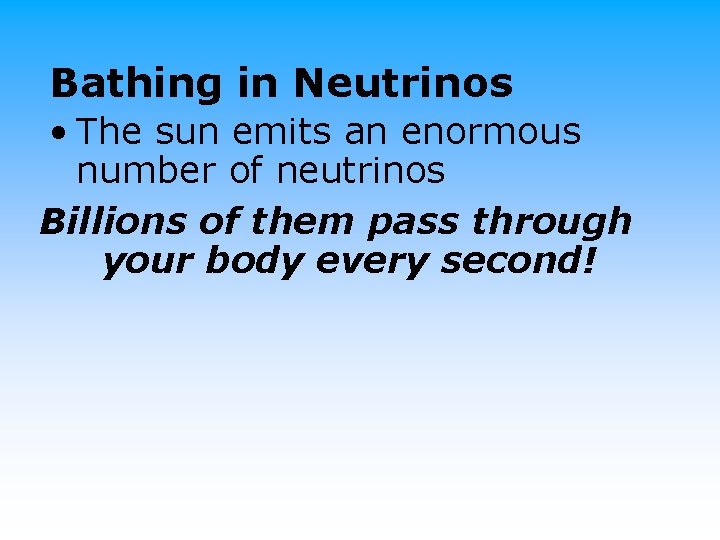
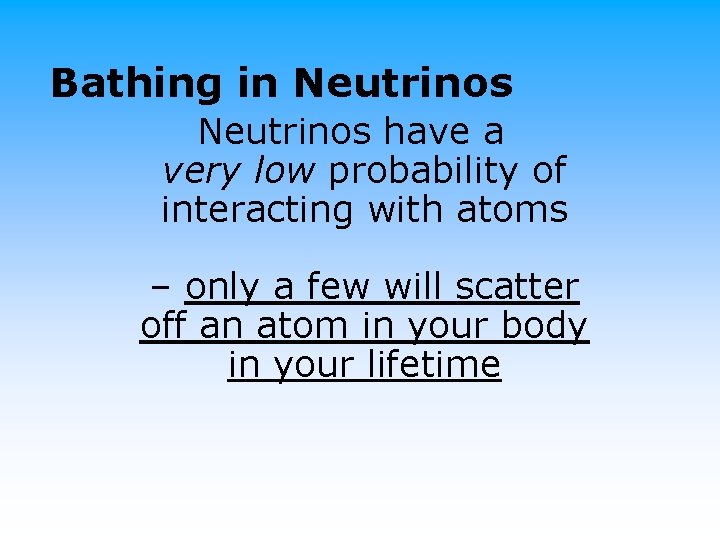
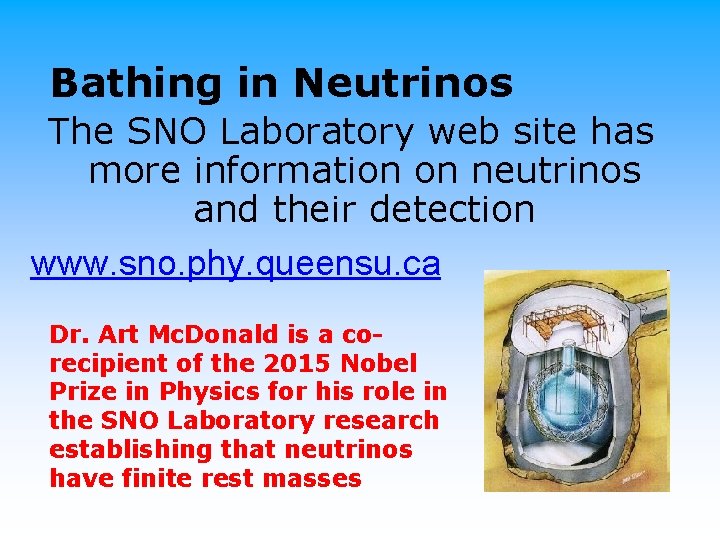
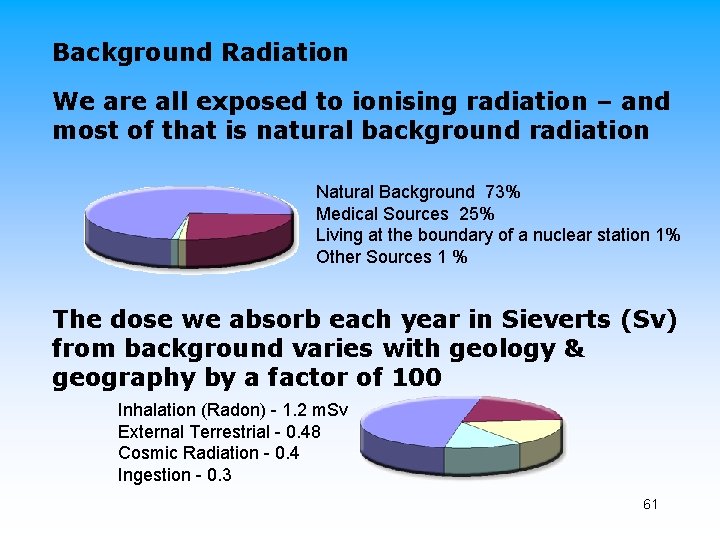
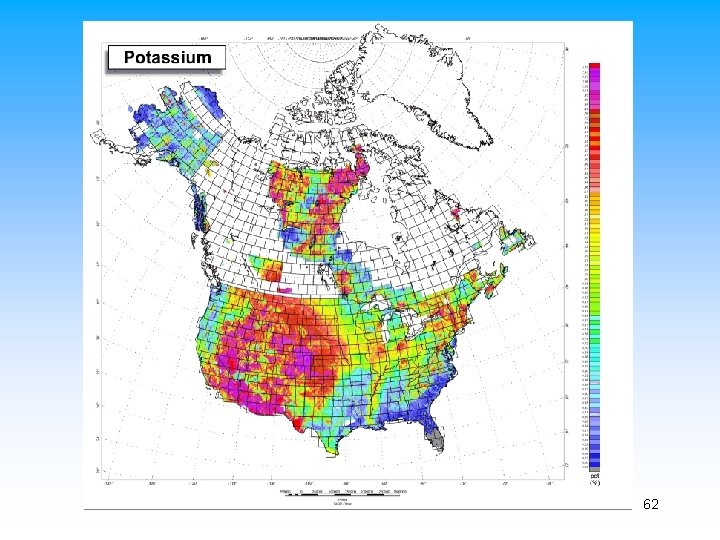
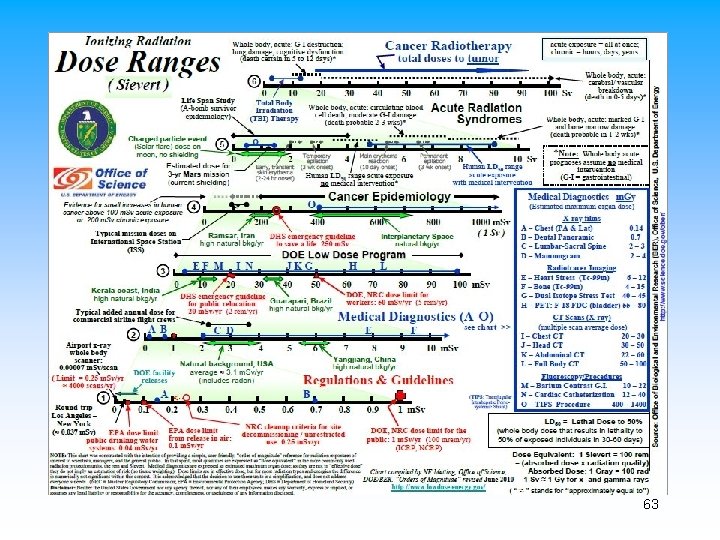
- Slides: 63
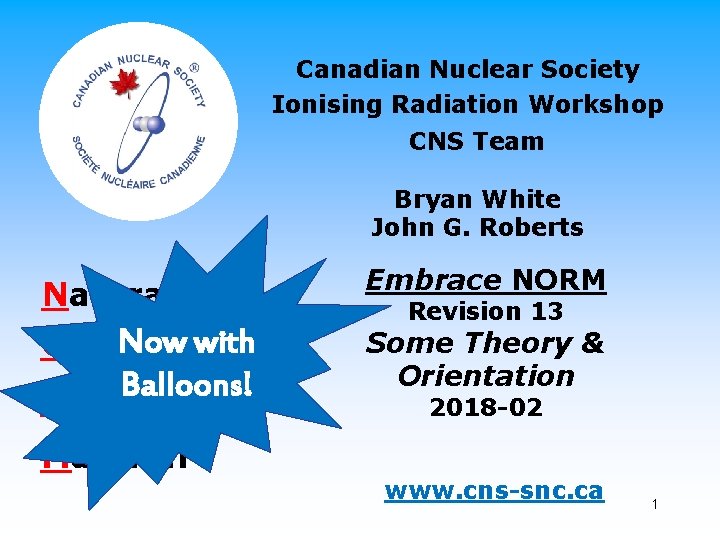
Canadian Nuclear Society Ionising Radiation Workshop CNS Team Bryan White John G. Roberts Naturally Embrace NORM Now with Occurring Some Theory & Orientation Balloons! Radioactive Revision 13 2018 -02 Material www. cns-snc. ca 1
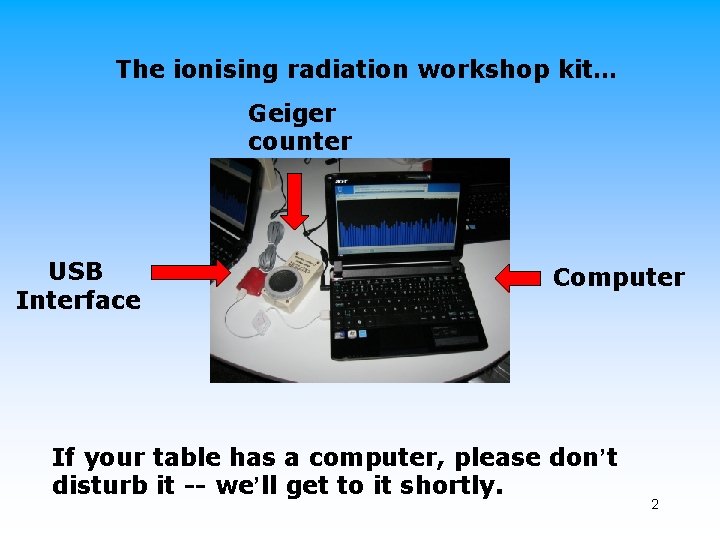
The ionising radiation workshop kit… Geiger counter USB Interface Computer If your table has a computer, please don’t disturb it -- we’ll get to it shortly. 2
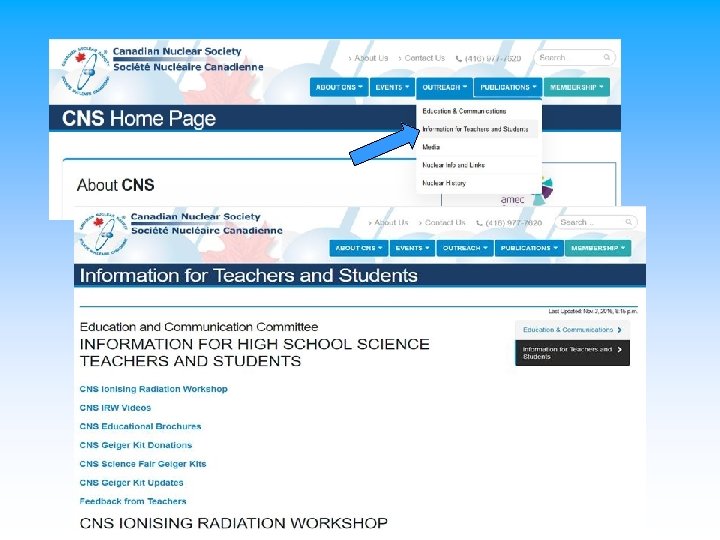
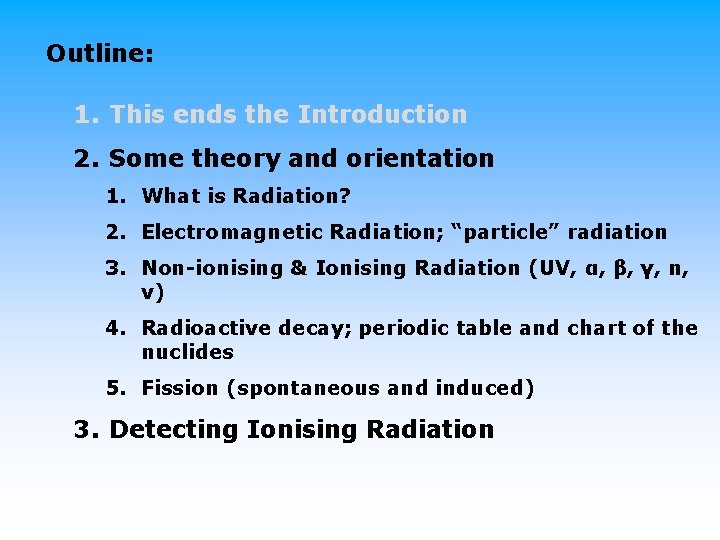
Outline: 1. This ends the Introduction 2. Some theory and orientation 1. What is Radiation? 2. Electromagnetic Radiation; “particle” radiation 3. Non-ionising & Ionising Radiation (UV, α, β, γ, n, ν) 4. Radioactive decay; periodic table and chart of the nuclides 5. Fission (spontaneous and induced) 3. Detecting Ionising Radiation
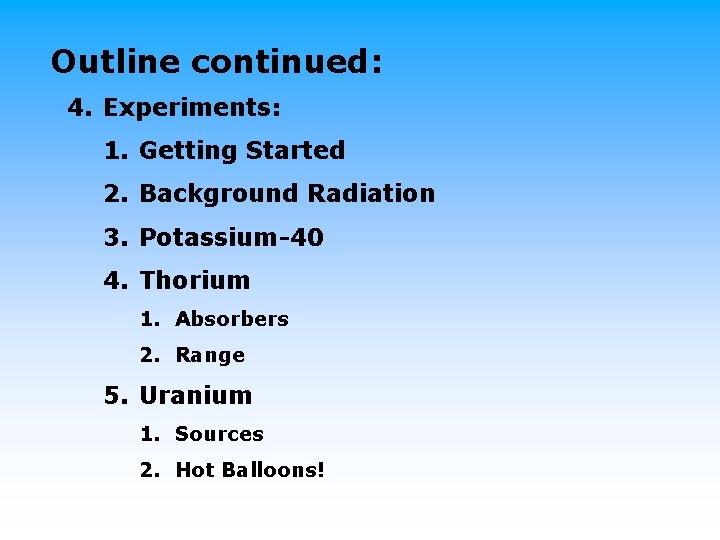
Outline continued: 4. Experiments: 1. Getting Started 2. Background Radiation 3. Potassium-40 4. Thorium 1. Absorbers 2. Range 5. Uranium 1. Sources 2. Hot Balloons!
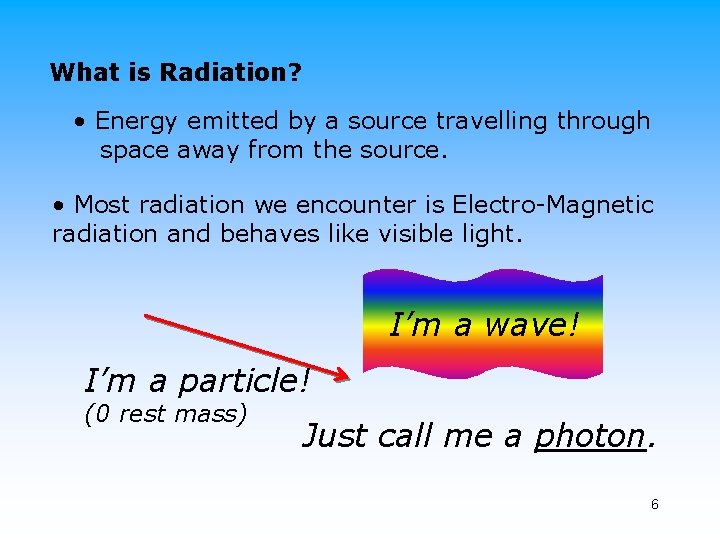
What is Radiation? • Energy emitted by a source travelling through space away from the source. • Most radiation we encounter is Electro-Magnetic radiation and behaves like visible light. I’m a wave! I’m a particle! (0 rest mass) Just call me a photon. 6
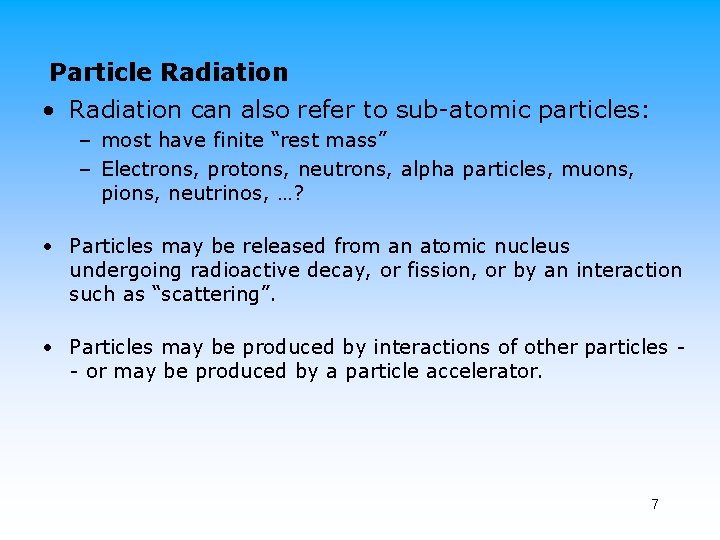
Particle Radiation • Radiation can also refer to sub-atomic particles: – most have finite “rest mass” – Electrons, protons, neutrons, alpha particles, muons, pions, neutrinos, …? • Particles may be released from an atomic nucleus undergoing radioactive decay, or fission, or by an interaction such as “scattering”. • Particles may be produced by interactions of other particles - or may be produced by a particle accelerator. 7
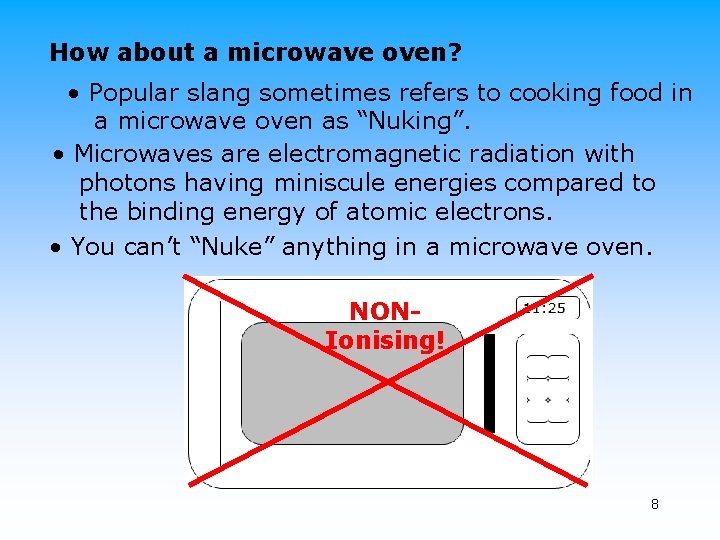
How about a microwave oven? • Popular slang sometimes refers to cooking food in a microwave oven as “Nuking”. • Microwaves are electromagnetic radiation with photons having miniscule energies compared to the binding energy of atomic electrons. • You can’t “Nuke” anything in a microwave oven. NONIonising! 8
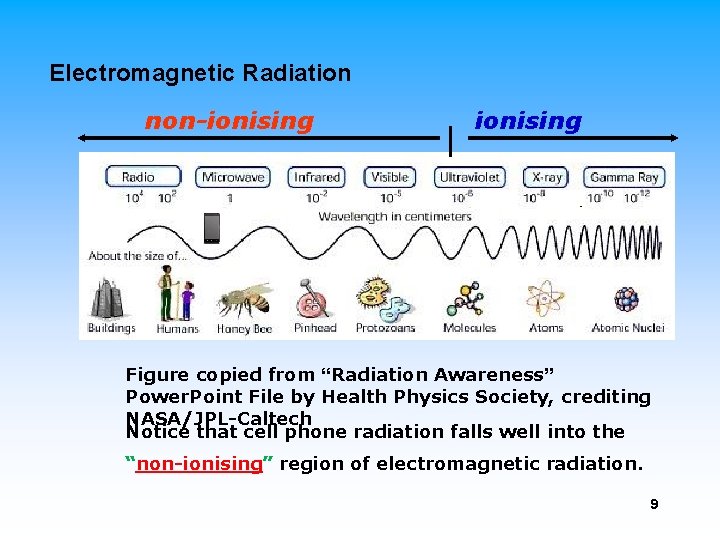
Electromagnetic Radiation non-ionising Figure copied from “Radiation Awareness” Power. Point File by Health Physics Society, crediting NASA/JPL-Caltech Notice that cell phone radiation falls well into the “non-ionising” region of electromagnetic radiation. 9
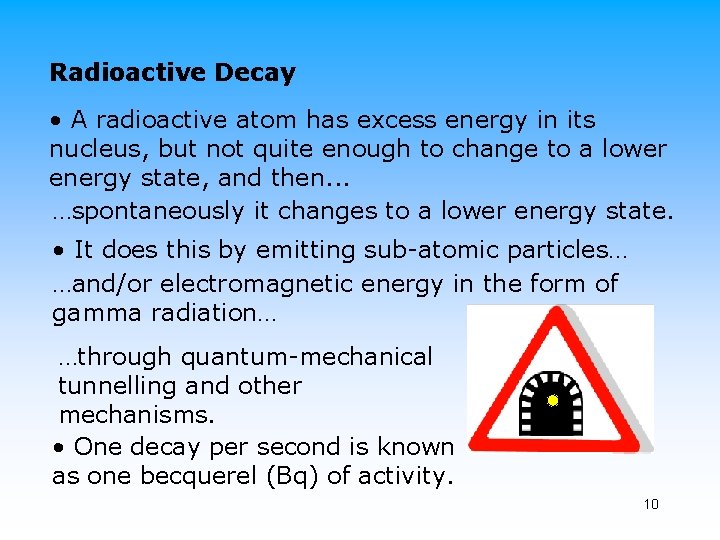
Radioactive Decay • A radioactive atom has excess energy in its nucleus, but not quite enough to change to a lower energy state, and then. . . …spontaneously it changes to a lower energy state. • It does this by emitting sub-atomic particles… …and/or electromagnetic energy in the form of gamma radiation… …through quantum-mechanical tunnelling and other mechanisms. • One decay per second is known as one becquerel (Bq) of activity. 10
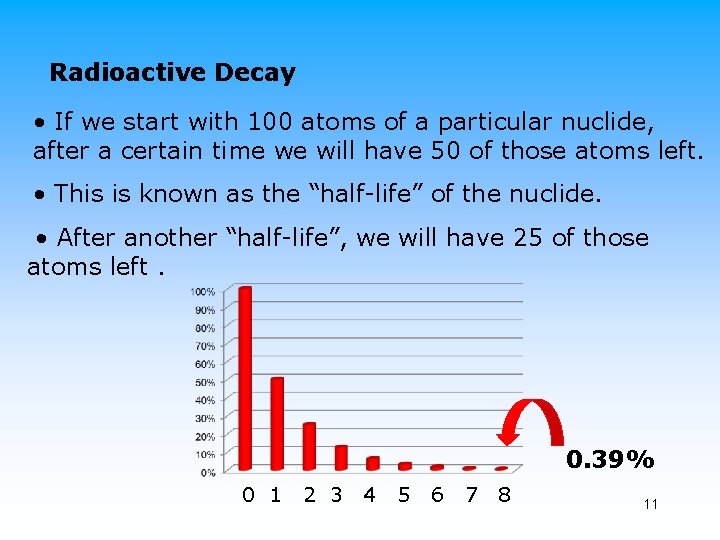
Radioactive Decay • If we start with 100 atoms of a particular nuclide, after a certain time we will have 50 of those atoms left. • This is known as the “half-life” of the nuclide. • After another “half-life”, we will have 25 of those atoms left. 0. 39% 0 1 2 3 4 5 6 7 8 11
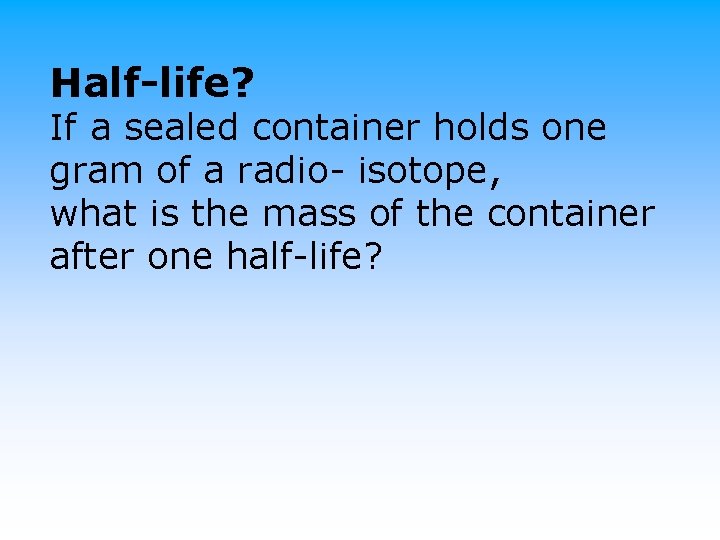
Half-life? If a sealed container holds one gram of a radio- isotope, what is the mass of the container after one half-life?
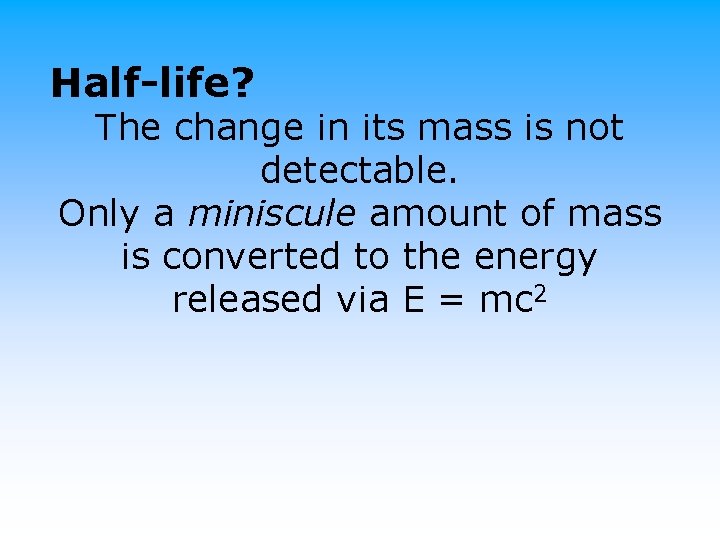
Half-life? The change in its mass is not detectable. Only a miniscule amount of mass is converted to the energy released via E = mc 2
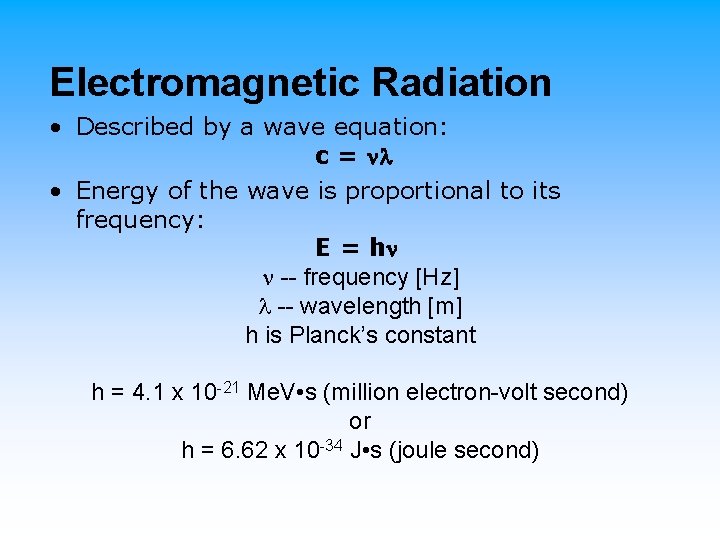
Electromagnetic Radiation • Described by a wave equation: c = • Energy of the wave is proportional to its frequency: E = h n -- frequency [Hz] -- wavelength [m] h is Planck’s constant h = 4. 1 x 10 -21 Me. V • s (million electron-volt second) or h = 6. 62 x 10 -34 J • s (joule second)
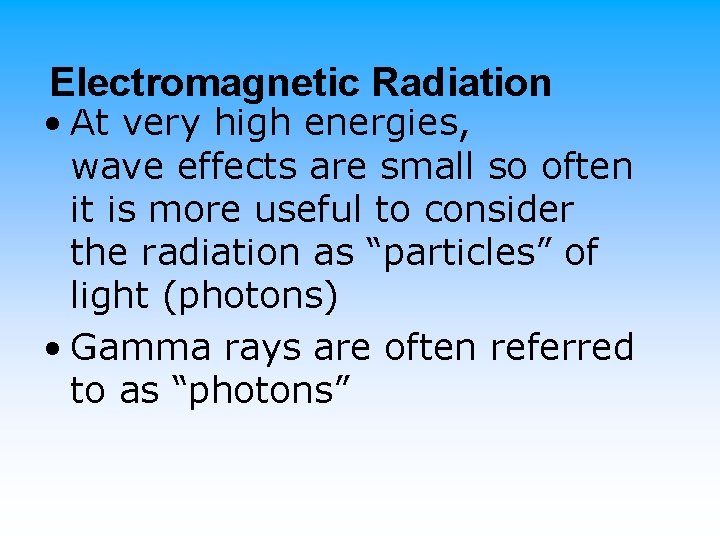
Electromagnetic Radiation • At very high energies, wave effects are small so often it is more useful to consider the radiation as “particles” of light (photons) • Gamma rays are often referred to as “photons”
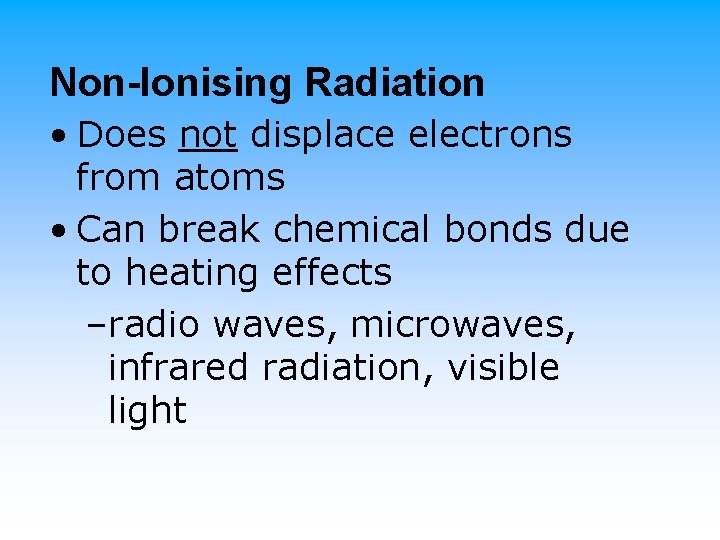
Non-Ionising Radiation • Does not displace electrons from atoms • Can break chemical bonds due to heating effects –radio waves, microwaves, infrared radiation, visible light
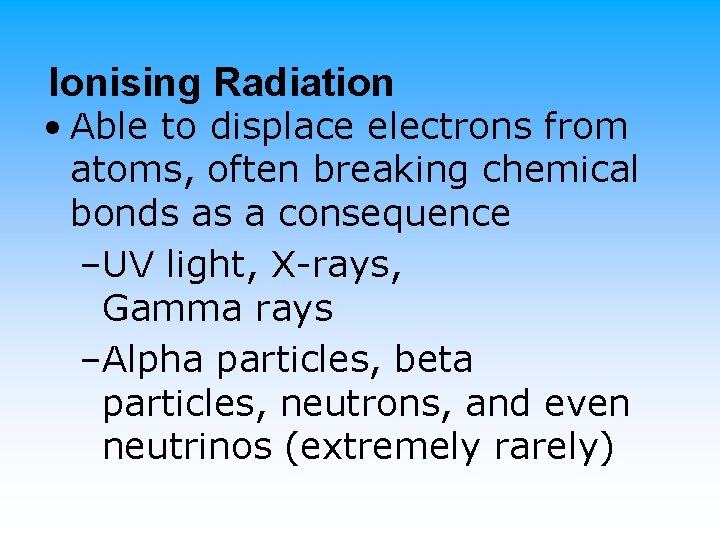
Ionising Radiation • Able to displace electrons from atoms, often breaking chemical bonds as a consequence –UV light, X-rays, Gamma rays –Alpha particles, beta particles, neutrons, and even neutrinos (extremely rarely)
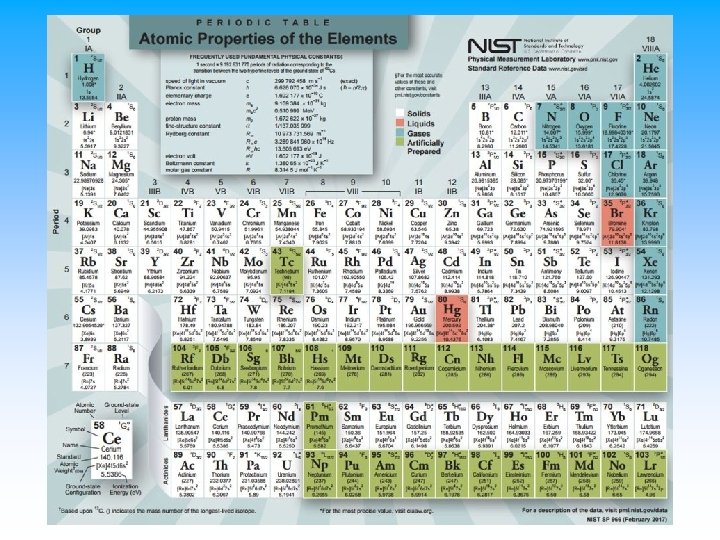
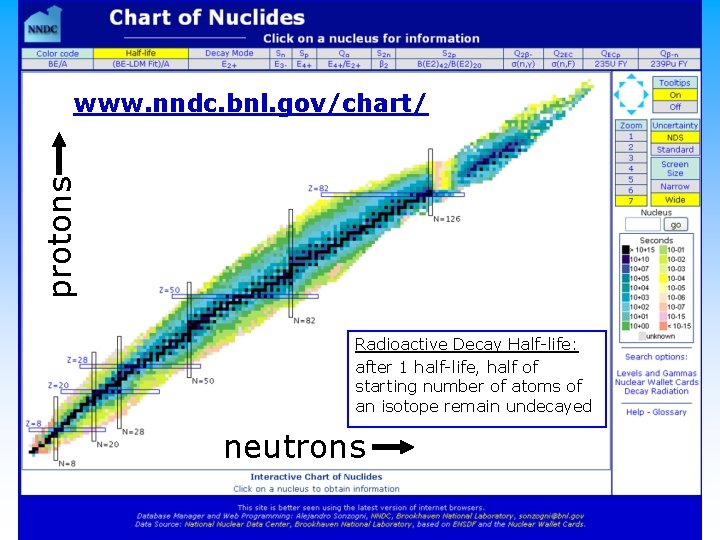
protons www. nndc. bnl. gov/chart/ Radioactive Decay Half-life: after 1 half-life, half of starting number of atoms of an isotope remain undecayed neutrons
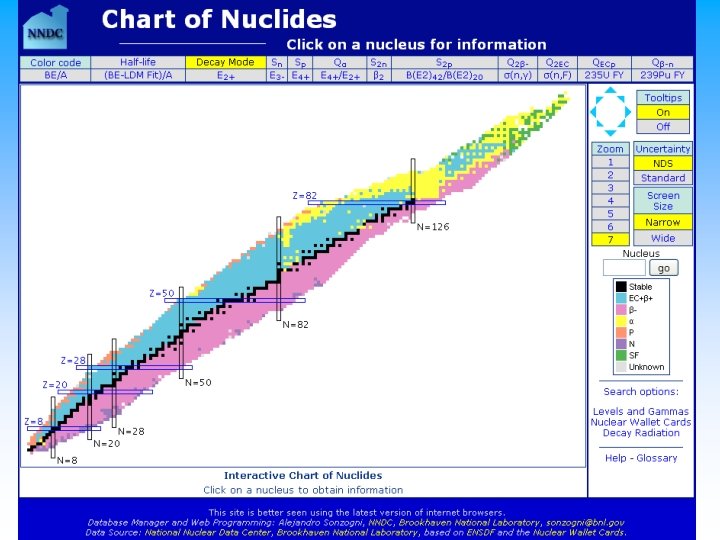
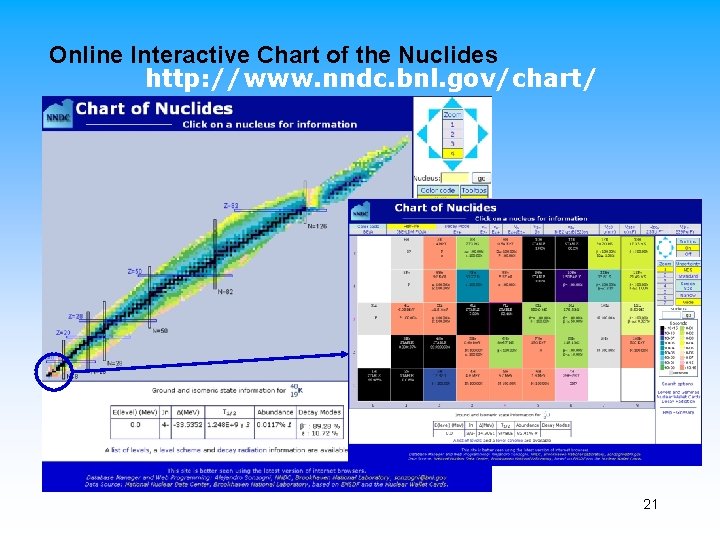
Online Interactive Chart of the Nuclides http: //www. nndc. bnl. gov/chart/ 21
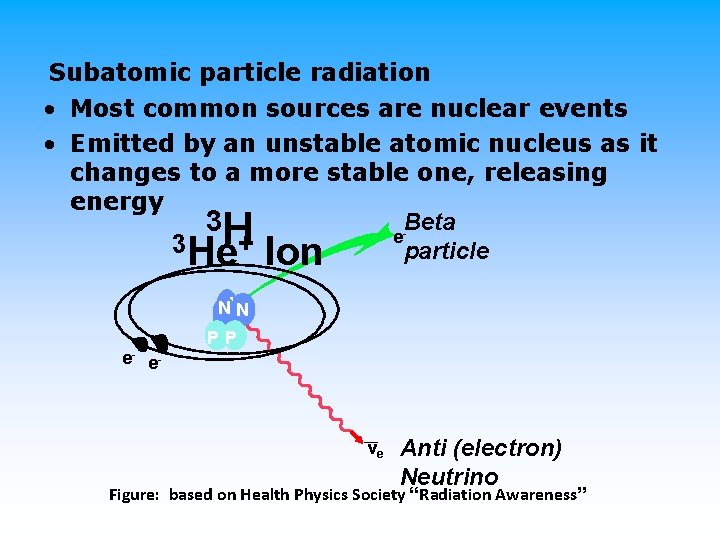
Subatomic particle radiation • Most common sources are nuclear events • Emitted by an unstable atomic nucleus as it changes to a more stable one, releasing energy Beta 3 e 3 + particle H He Ion NN N P PP e- e- νe Anti (electron) Neutrino Figure: based on Health Physics Society “Radiation Awareness”
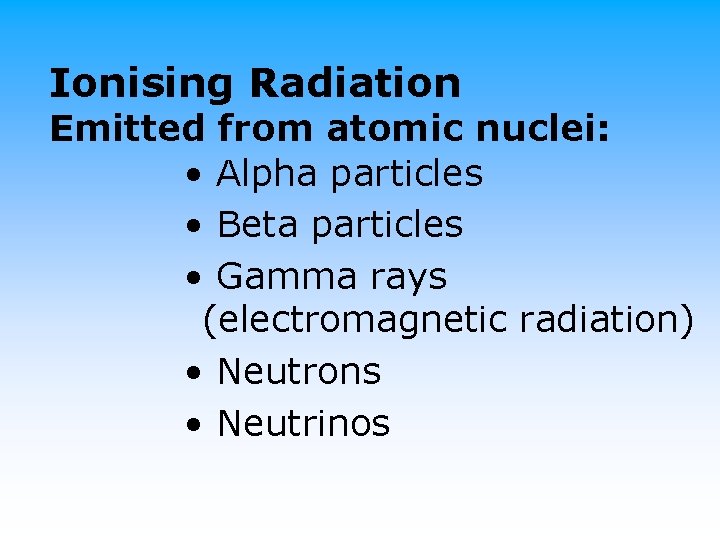
Ionising Radiation Emitted from atomic nuclei: • Alpha particles • Beta particles • Gamma rays (electromagnetic radiation) • Neutrons • Neutrinos
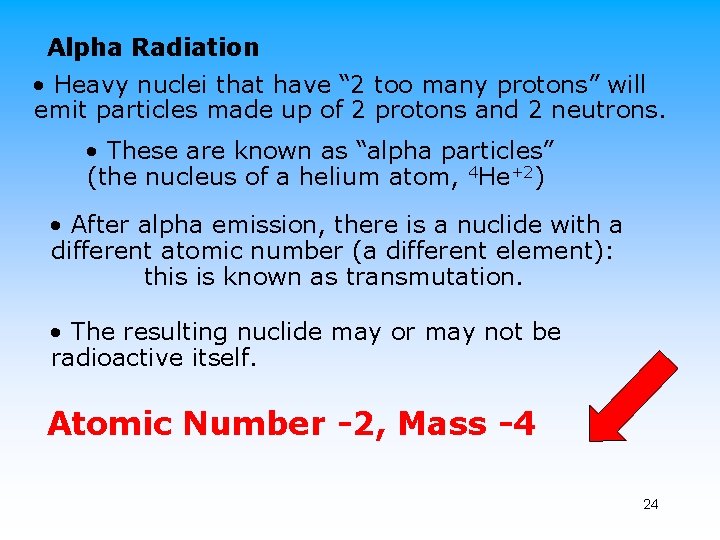
Alpha Radiation • Heavy nuclei that have “ 2 too many protons” will emit particles made up of 2 protons and 2 neutrons. • These are known as “alpha particles” (the nucleus of a helium atom, 4 He+2) • After alpha emission, there is a nuclide with a different atomic number (a different element): this is known as transmutation. • The resulting nuclide may or may not be radioactive itself. Atomic Number -2, Mass -4 24
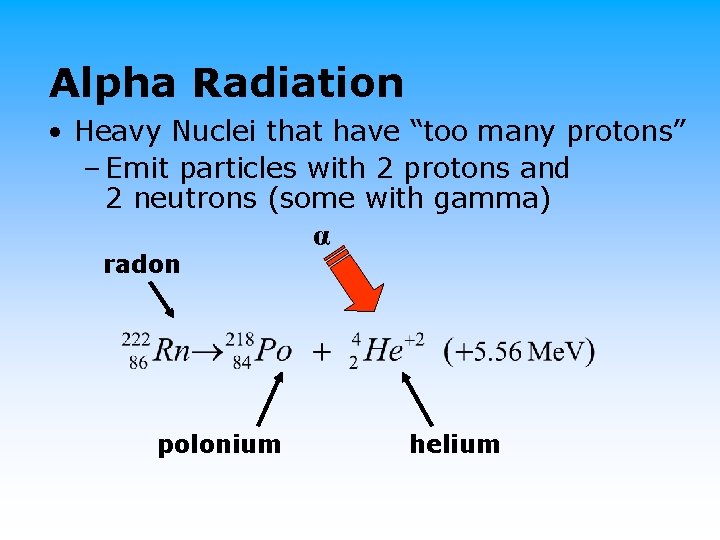
Alpha Radiation • Heavy Nuclei that have “too many protons” – Emit particles with 2 protons and 2 neutrons (some with gamma) radon polonium α helium
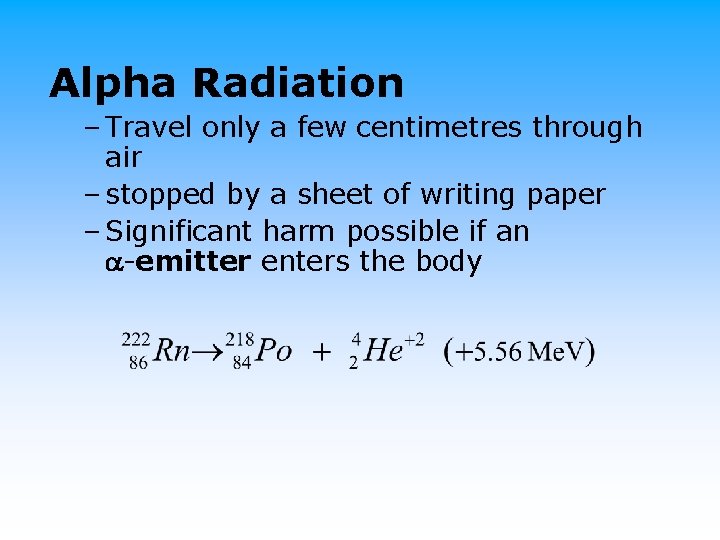
Alpha Radiation – Travel only a few centimetres through air – stopped by a sheet of writing paper – Significant harm possible if an -emitter enters the body
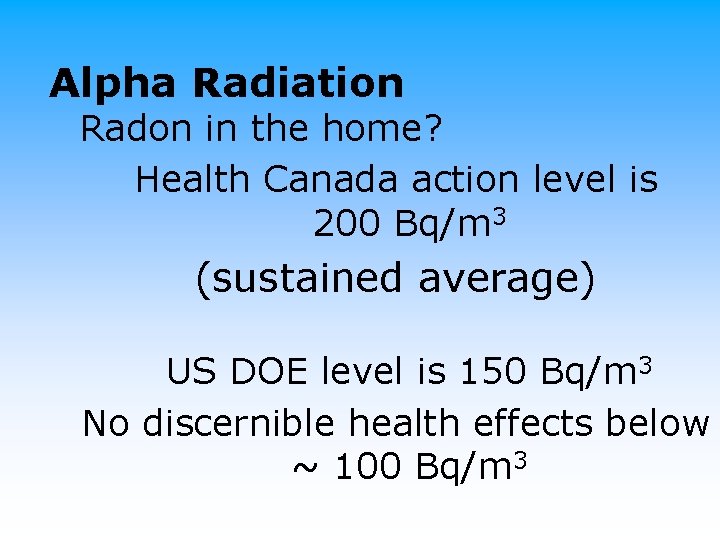
Alpha Radiation Radon in the home? Health Canada action level is 200 Bq/m 3 (sustained average) US DOE level is 150 Bq/m 3 No discernible health effects below ~ 100 Bq/m 3
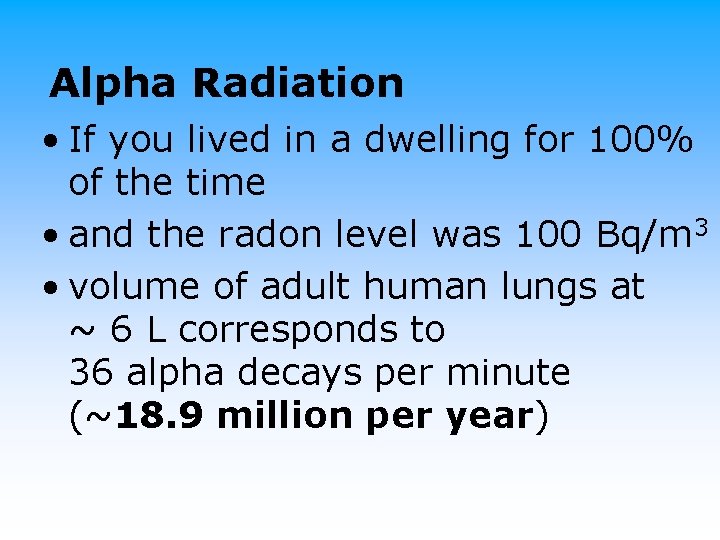
Alpha Radiation • If you lived in a dwelling for 100% of the time • and the radon level was 100 Bq/m 3 • volume of adult human lungs at ~ 6 L corresponds to 36 alpha decays per minute (~18. 9 million per year)
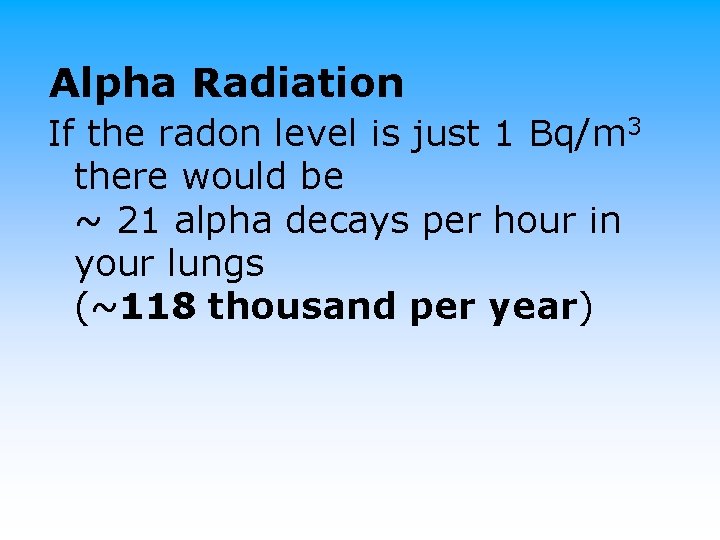
Alpha Radiation If the radon level is just 1 Bq/m 3 there would be ~ 21 alpha decays per hour in your lungs (~118 thousand per year)
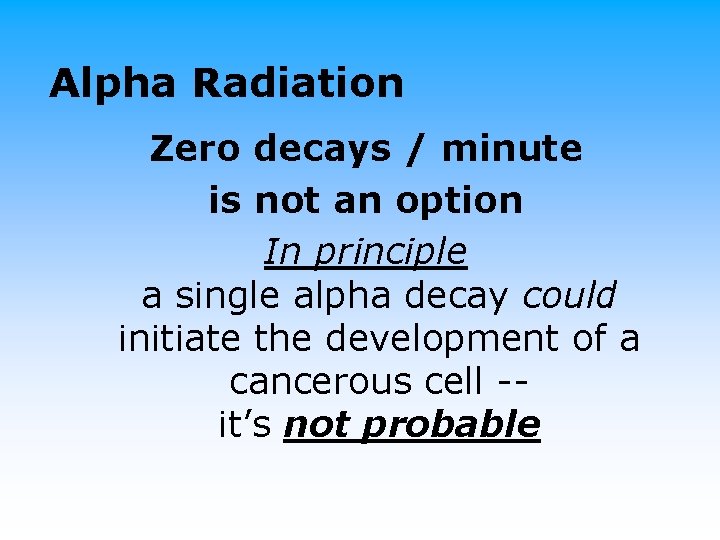
Alpha Radiation Zero decays / minute is not an option In principle a single alpha decay could initiate the development of a cancerous cell -it’s not probable
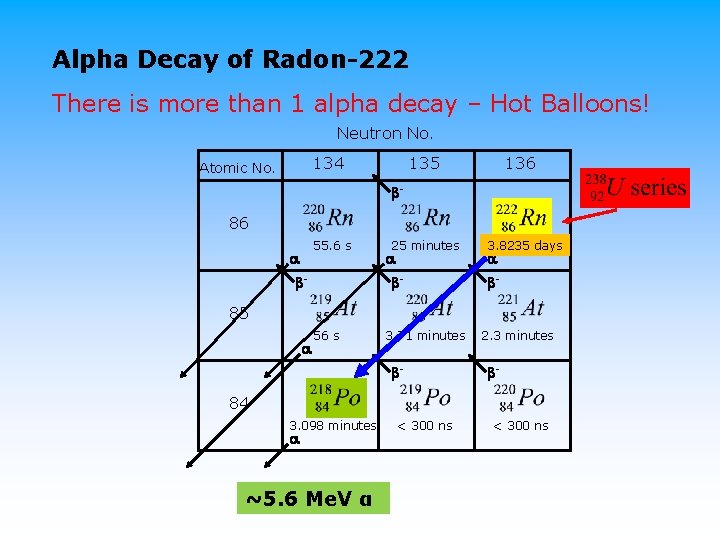
Alpha Decay of Radon-222 There is more than 1 alpha decay – Hot Balloons! Neutron No. 134 Atomic No. 135 136 - 86 55. 6 s - 25 minutes 3. 8235 days - - 85 56 s 3. 71 minutes - 2. 3 minutes - 84 3. 098 minutes ~5. 6 Me. V α < 300 ns
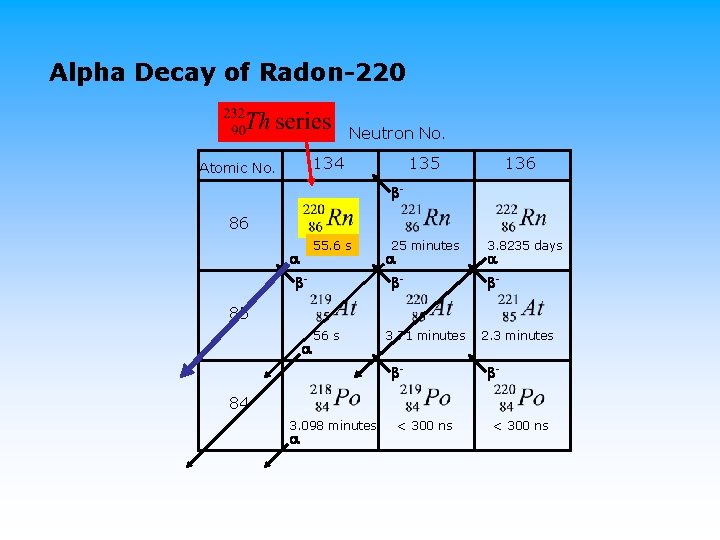
Alpha Decay of Radon-220 Neutron No. 134 Atomic No. 135 136 - 86 55. 6 s - 25 minutes 3. 8235 days - - 85 56 s 3. 71 minutes - 2. 3 minutes - 84 3. 098 minutes < 300 ns
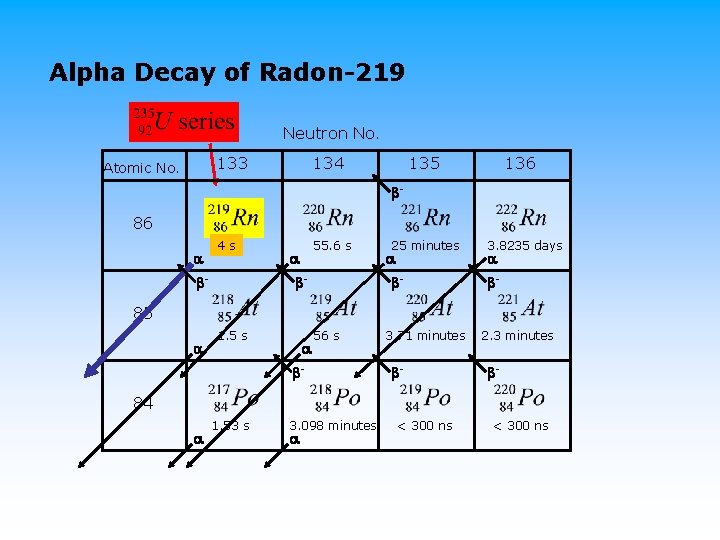
Alpha Decay of Radon-219 Neutron No. 133 Atomic No. 134 135 136 - 86 4 s - 55. 6 s - 25 minutes 3. 8235 days - - 85 1. 5 s 56 s - 3. 71 minutes - 2. 3 minutes - 84 1. 53 s 3. 098 minutes < 300 ns
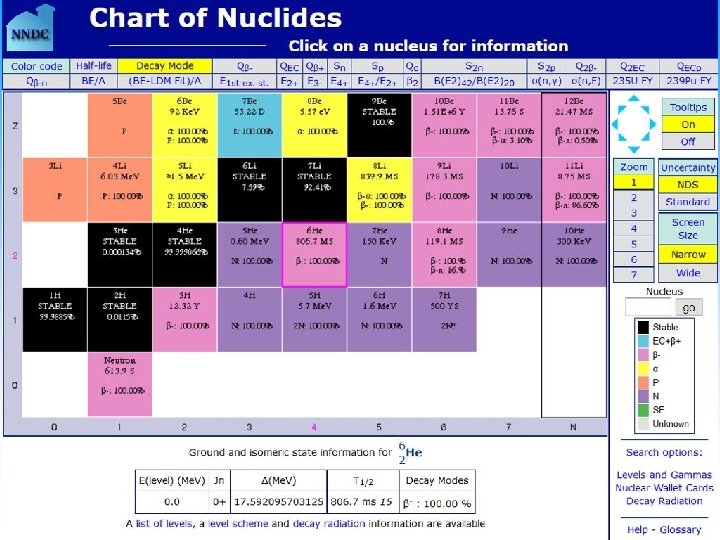
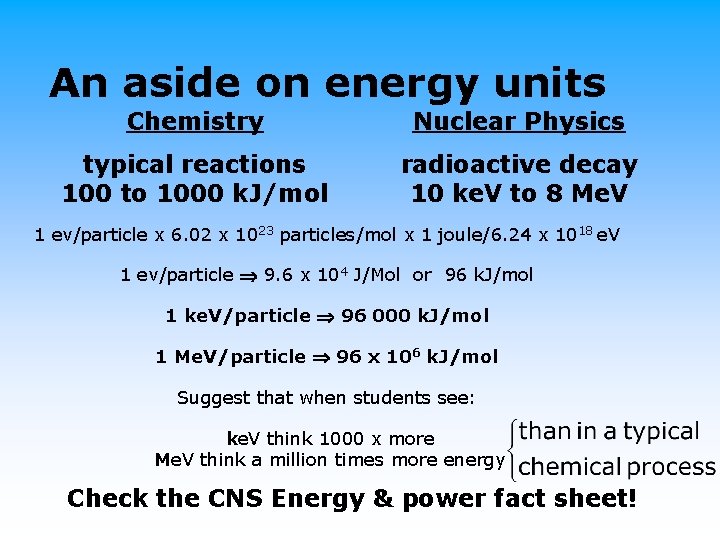
An aside on energy units Chemistry Nuclear Physics typical reactions 100 to 1000 k. J/mol radioactive decay 10 ke. V to 8 Me. V 1 ev/particle x 6. 02 x 1023 particles/mol x 1 joule/6. 24 x 1018 e. V 1 ev/particle 9. 6 x 104 J/Mol or 96 k. J/mol 1 ke. V/particle 96 000 k. J/mol 1 Me. V/particle 96 x 106 k. J/mol Suggest that when students see: ke. V think 1000 x more Me. V think a million times more energy Check the CNS Energy & power fact sheet!
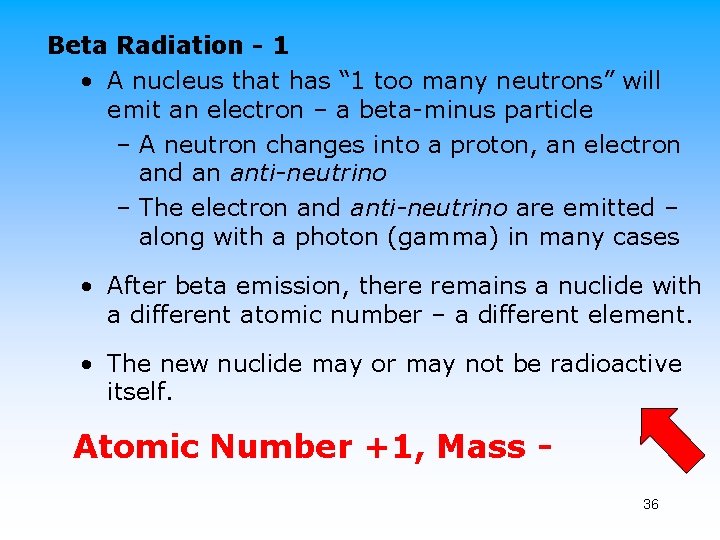
Beta Radiation - 1 • A nucleus that has “ 1 too many neutrons” will emit an electron – a beta-minus particle – A neutron changes into a proton, an electron and an anti-neutrino – The electron and anti-neutrino are emitted – along with a photon (gamma) in many cases • After beta emission, there remains a nuclide with a different atomic number – a different element. • The new nuclide may or may not be radioactive itself. Atomic Number +1, Mass 36
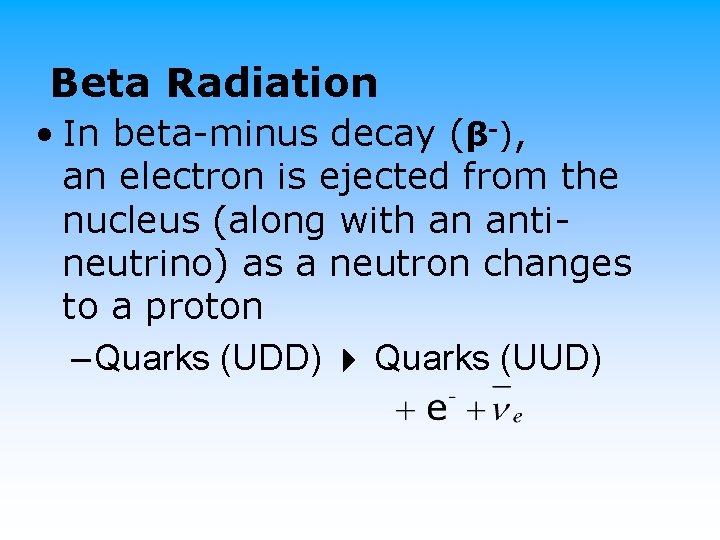
Beta Radiation • In beta-minus decay (β-), an electron is ejected from the nucleus (along with an antineutrino) as a neutron changes to a proton – Quarks (UDD) 4 Quarks (UUD)
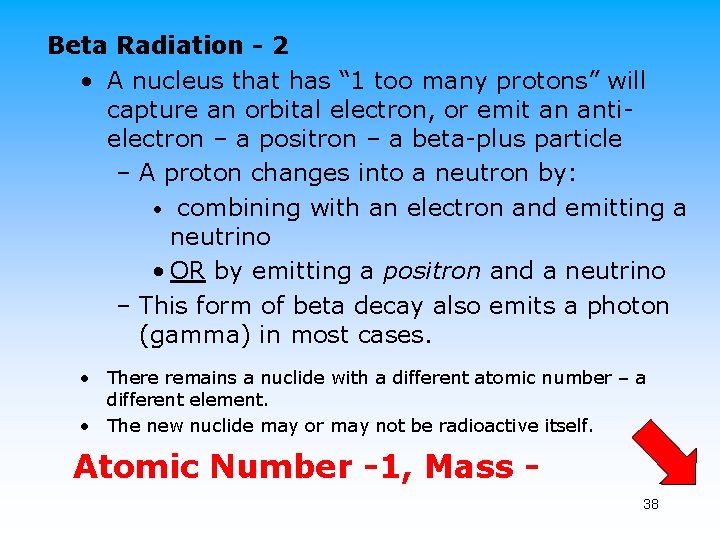
Beta Radiation - 2 • A nucleus that has “ 1 too many protons” will capture an orbital electron, or emit an antielectron – a positron – a beta-plus particle – A proton changes into a neutron by: • combining with an electron and emitting a neutrino • OR by emitting a positron and a neutrino – This form of beta decay also emits a photon (gamma) in most cases. • There remains a nuclide with a different atomic number – a different element. • The new nuclide may or may not be radioactive itself. Atomic Number -1, Mass 38
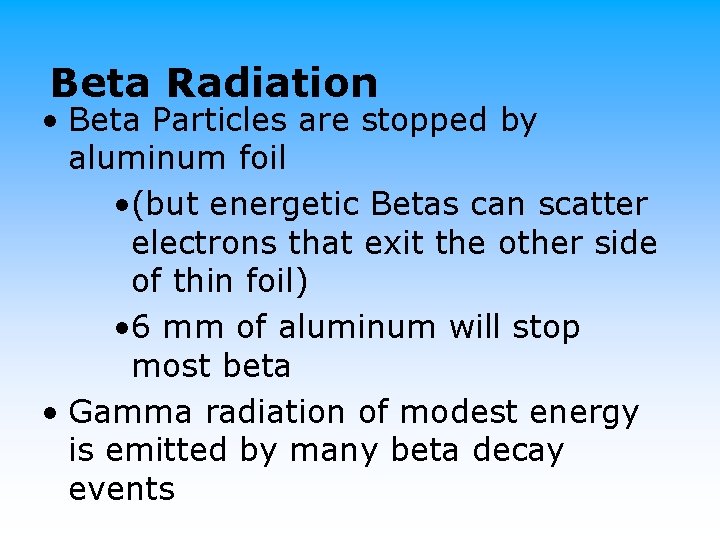
Beta Radiation • Beta Particles are stopped by aluminum foil • (but energetic Betas can scatter electrons that exit the other side of thin foil) • 6 mm of aluminum will stop most beta • Gamma radiation of modest energy is emitted by many beta decay events
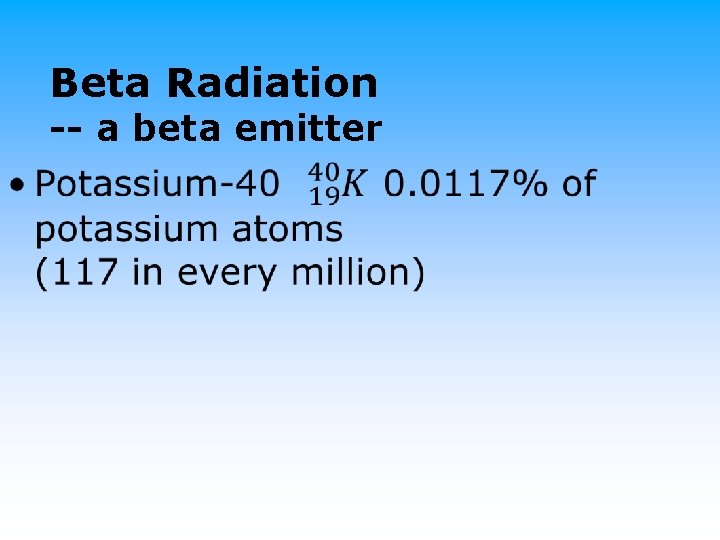
Beta Radiation -- a beta emitter •
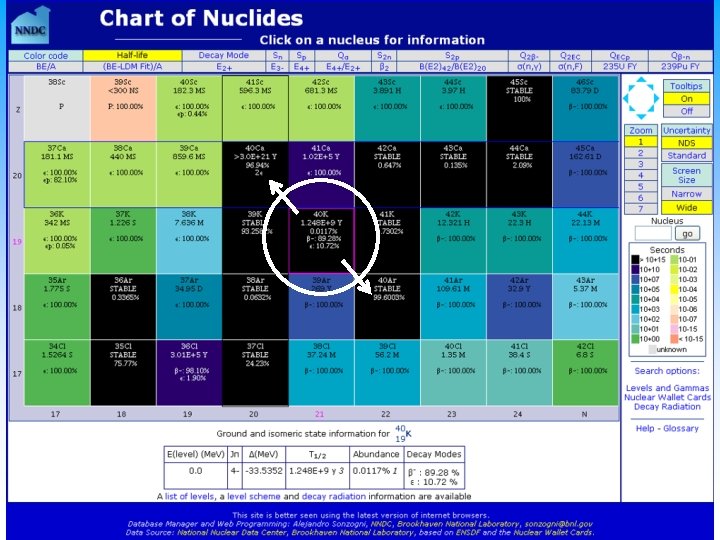
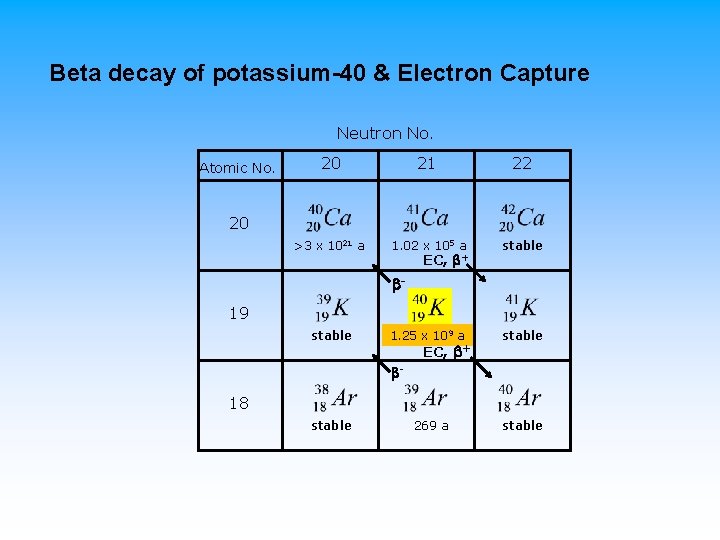
Beta decay of potassium-40 & Electron Capture Neutron No. Atomic No. 20 21 22 >3 x 1021 a 1. 02 x 105 a stable 20 EC, + 19 stable 1. 25 x 109 a EC, + stable - 18 stable 269 a stable
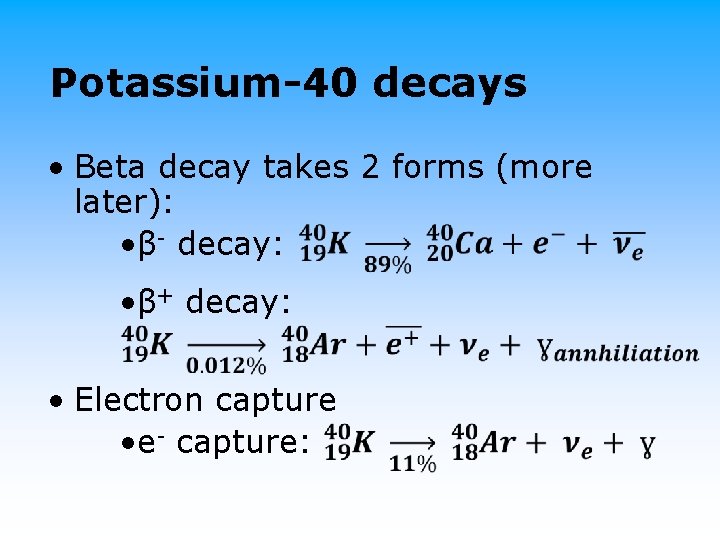
Potassium-40 decays • Beta decay takes 2 forms (more later): • β- decay: • β+ decay: • Electron capture • e- capture:
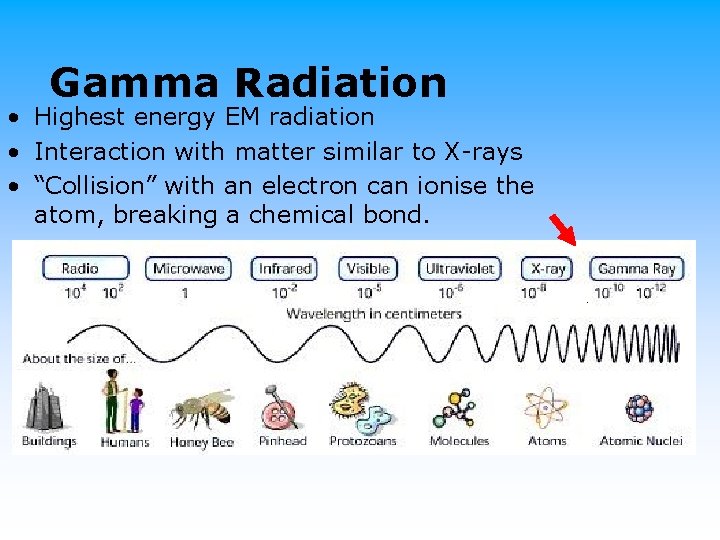
Gamma Radiation • Highest energy EM radiation • Interaction with matter similar to X-rays • “Collision” with an electron can ionise the atom, breaking a chemical bond.
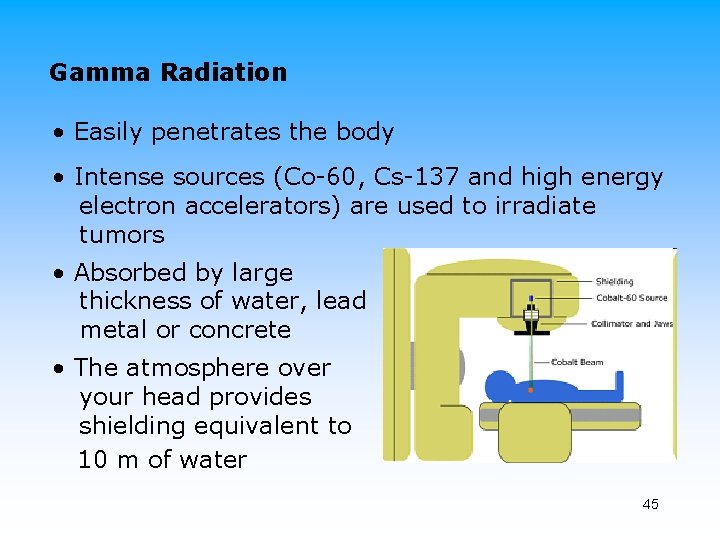
Gamma Radiation • Easily penetrates the body • Intense sources (Co-60, Cs-137 and high energy electron accelerators) are used to irradiate tumors • Absorbed by large thickness of water, lead metal or concrete • The atmosphere over your head provides shielding equivalent to 10 m of water 45
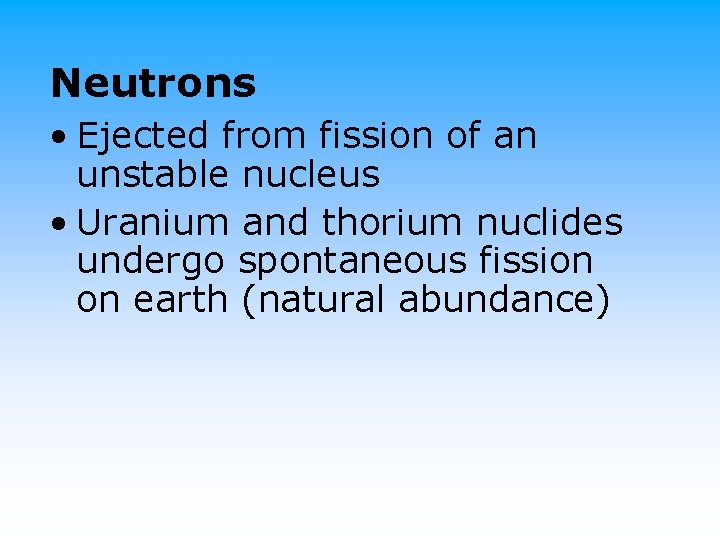
Neutrons • Ejected from fission of an unstable nucleus • Uranium and thorium nuclides undergo spontaneous fission on earth (natural abundance)
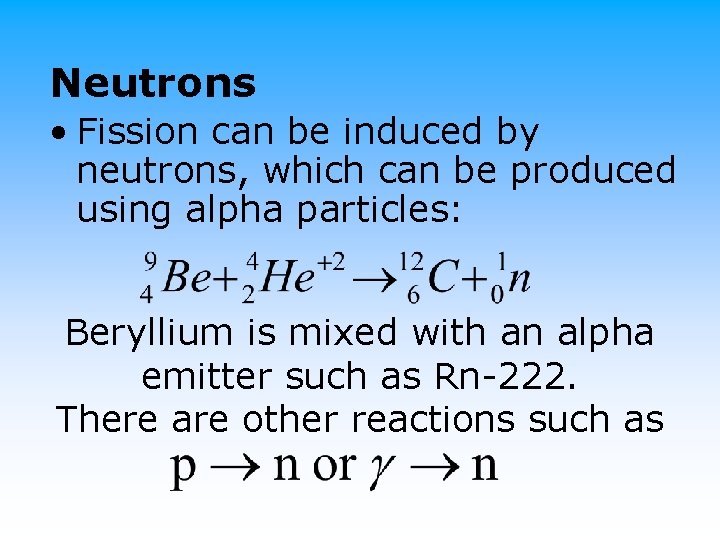
Neutrons • Fission can be induced by neutrons, which can be produced using alpha particles: Beryllium is mixed with an alpha emitter such as Rn-222. There are other reactions such as
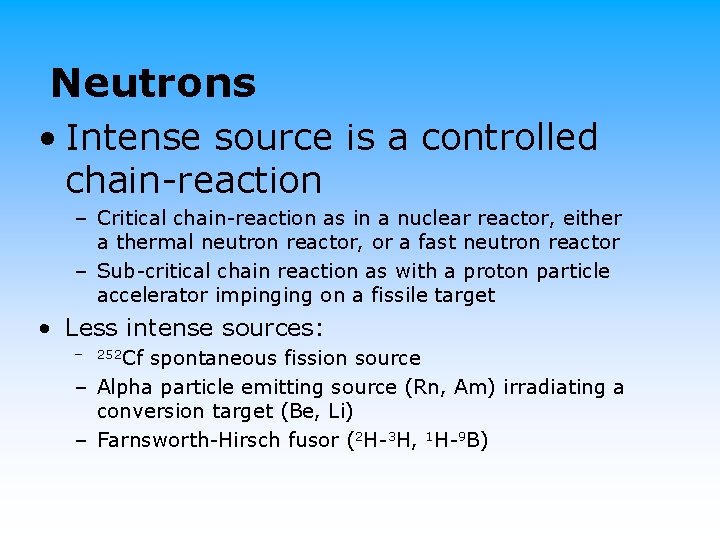
Neutrons • Intense source is a controlled chain-reaction – Critical chain-reaction as in a nuclear reactor, either a thermal neutron reactor, or a fast neutron reactor – Sub-critical chain reaction as with a proton particle accelerator impinging on a fissile target • Less intense sources: spontaneous fission source – Alpha particle emitting source (Rn, Am) irradiating a conversion target (Be, Li) – Farnsworth-Hirsch fusor (2 H-3 H, 1 H-9 B) – 252 Cf
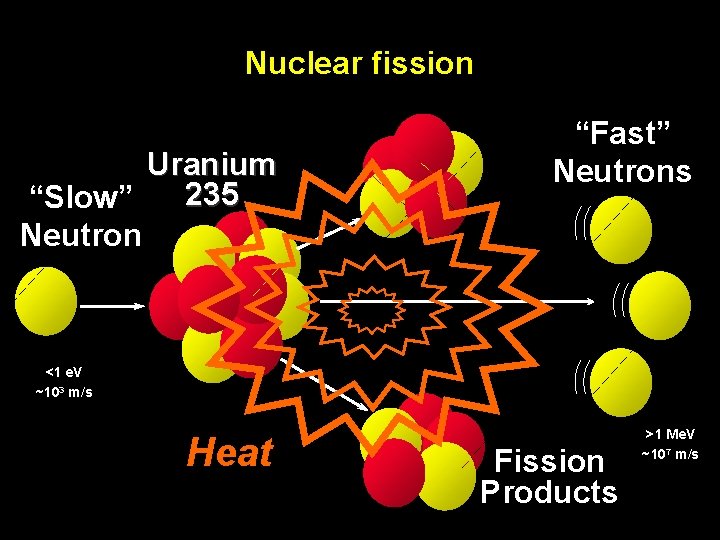
Nuclear fission Uranium 235 “Slow” Neutron “Fast” Neutrons <1 e. V ~103 m/s Heat Fission Products >1 Me. V ~107 m/s
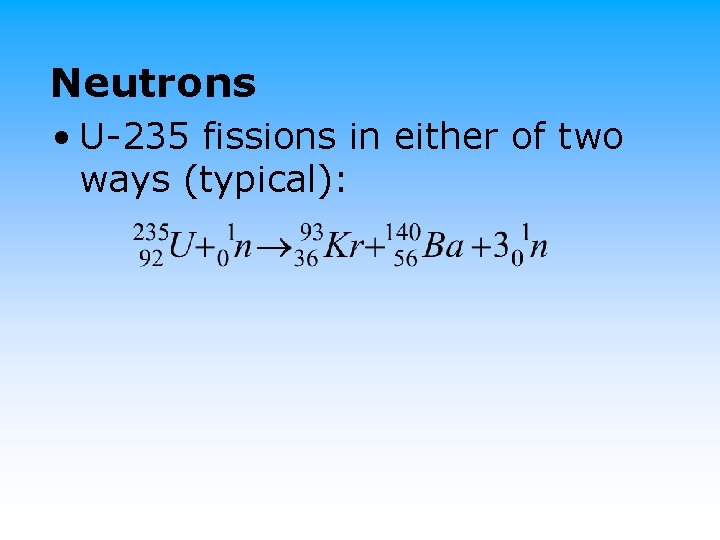
Neutrons • U-235 fissions in either of two ways (typical):
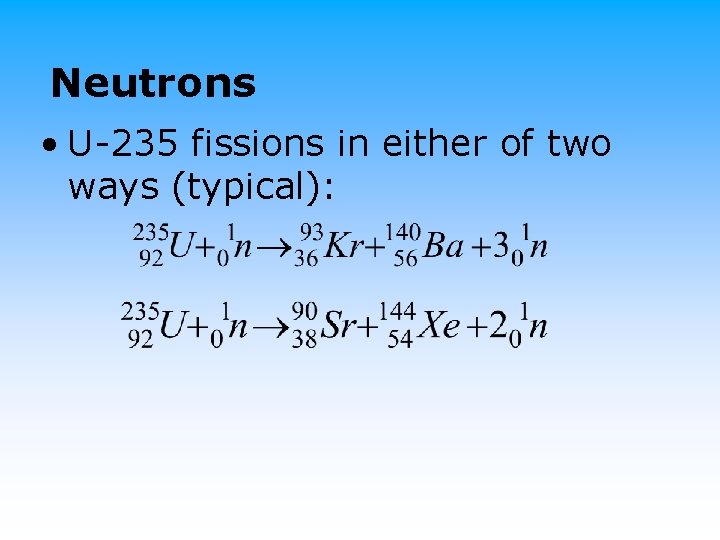
Neutrons • U-235 fissions in either of two ways (typical):
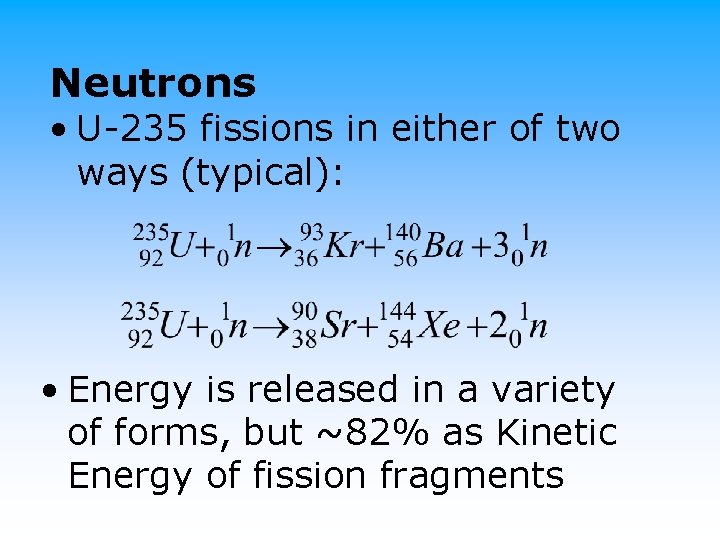
Neutrons • U-235 fissions in either of two ways (typical): • Energy is released in a variety of forms, but ~82% as Kinetic Energy of fission fragments
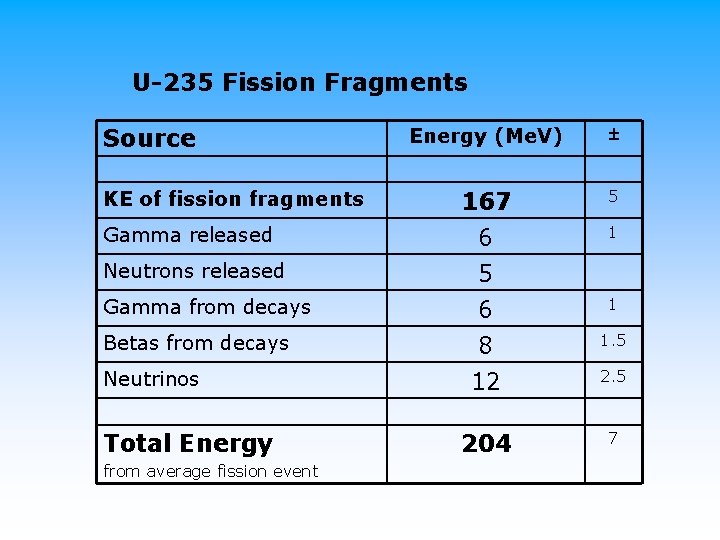
U-235 Fission Fragments Energy (Me. V) 167 5 Gamma released 6 1 Neutrons released 5 Gamma from decays 6 1 Betas from decays 8 1. 5 12 2. 5 204 7 Source KE of fission fragments Neutrinos Total Energy from average fission event
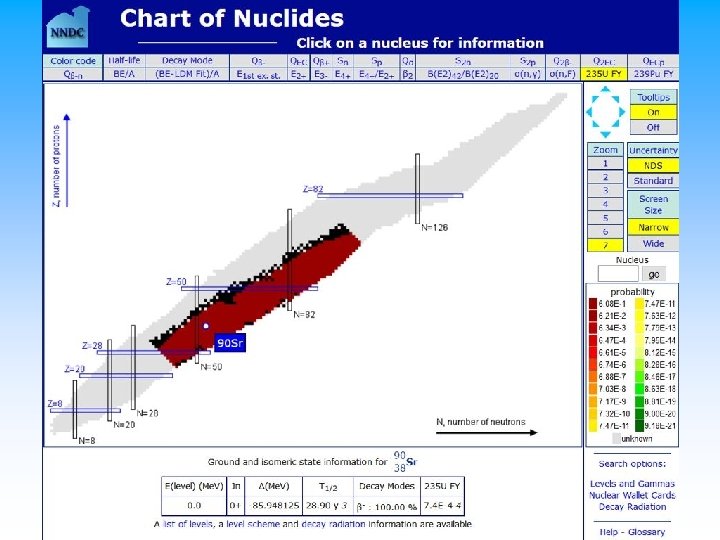
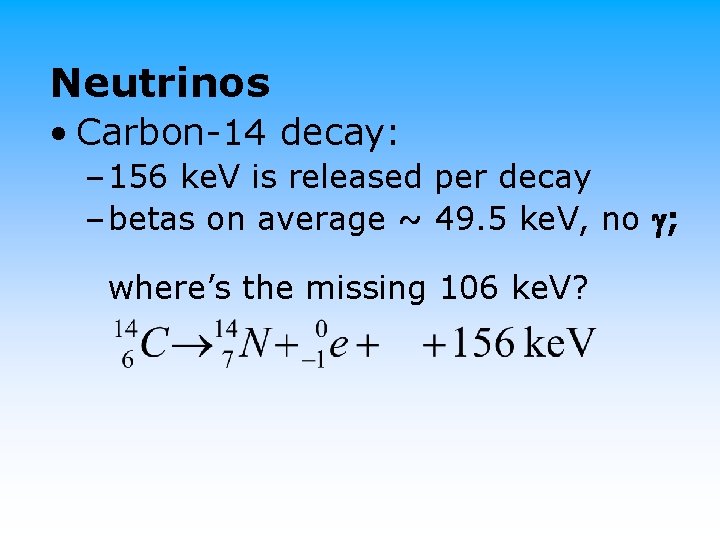
Neutrinos • Carbon-14 decay: – 156 ke. V is released per decay – betas on average ~ 49. 5 ke. V, no ; where’s the missing 106 ke. V?
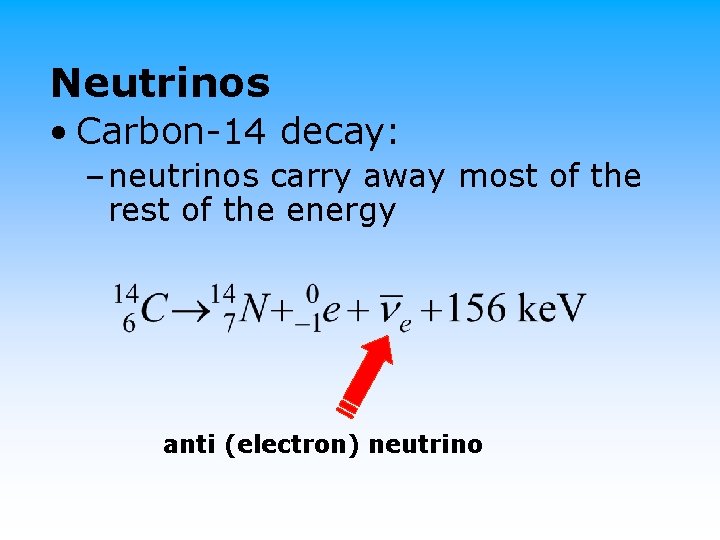
Neutrinos • Carbon-14 decay: – neutrinos carry away most of the rest of the energy anti (electron) neutrino
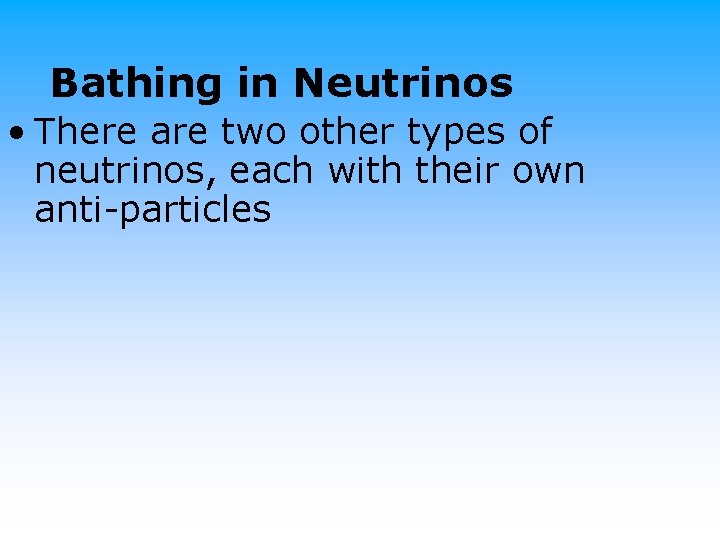
Bathing in Neutrinos • There are two other types of neutrinos, each with their own anti-particles
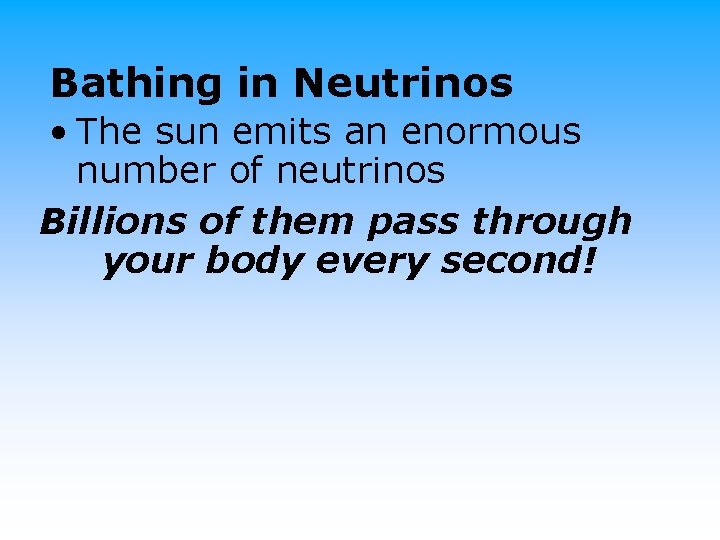
Bathing in Neutrinos • The sun emits an enormous number of neutrinos Billions of them pass through your body every second!
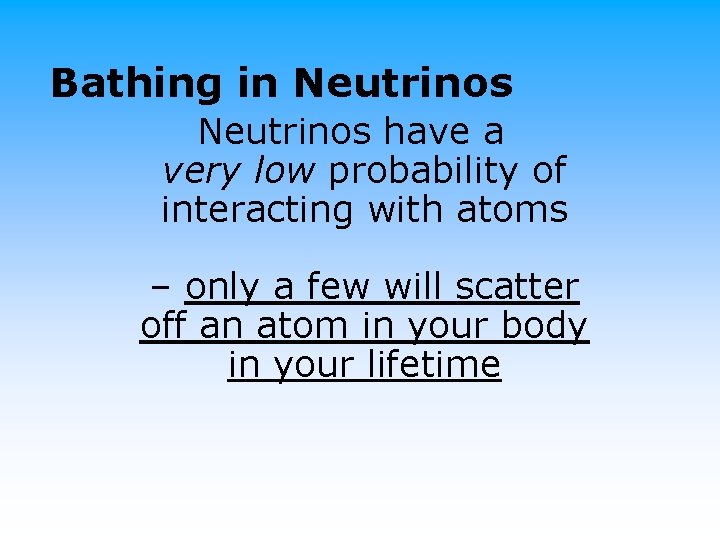
Bathing in Neutrinos have a very low probability of interacting with atoms – only a few will scatter off an atom in your body in your lifetime
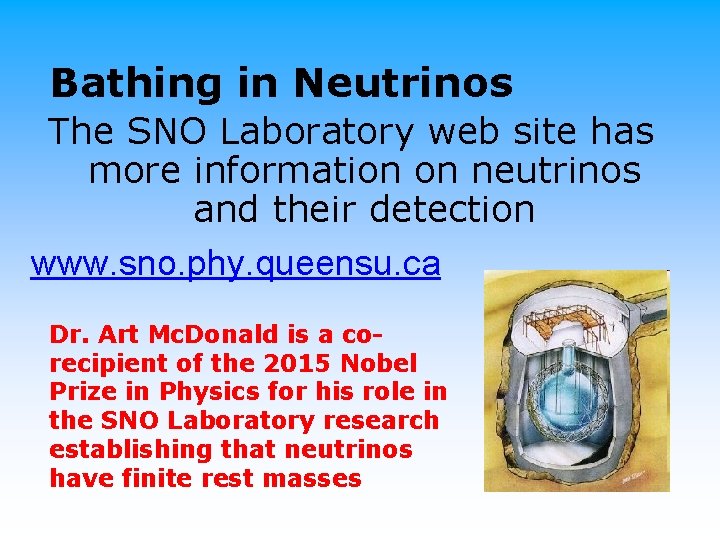
Bathing in Neutrinos The SNO Laboratory web site has more information on neutrinos and their detection www. sno. phy. queensu. ca Dr. Art Mc. Donald is a corecipient of the 2015 Nobel Prize in Physics for his role in the SNO Laboratory research establishing that neutrinos have finite rest masses
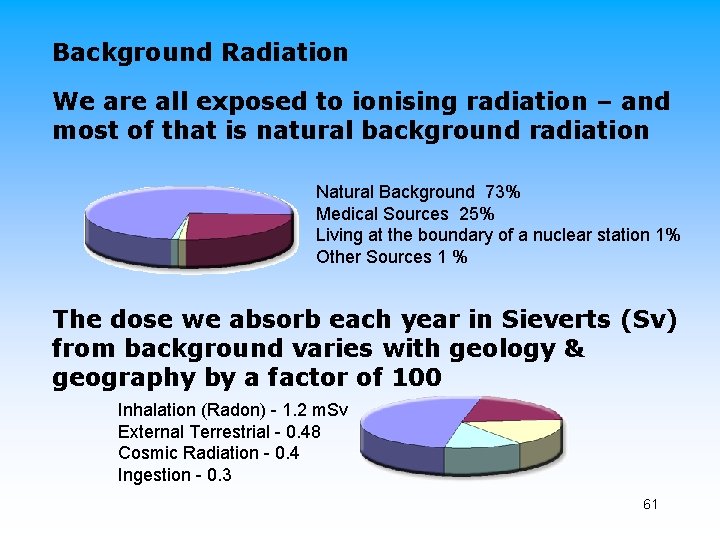
Background Radiation We are all exposed to ionising radiation – and most of that is natural background radiation Natural Background 73% Medical Sources 25% Living at the boundary of a nuclear station 1% Other Sources 1 % The dose we absorb each year in Sieverts (Sv) from background varies with geology & geography by a factor of 100 Inhalation (Radon) - 1. 2 m. Sv External Terrestrial - 0. 48 Cosmic Radiation - 0. 4 Ingestion - 0. 3 61
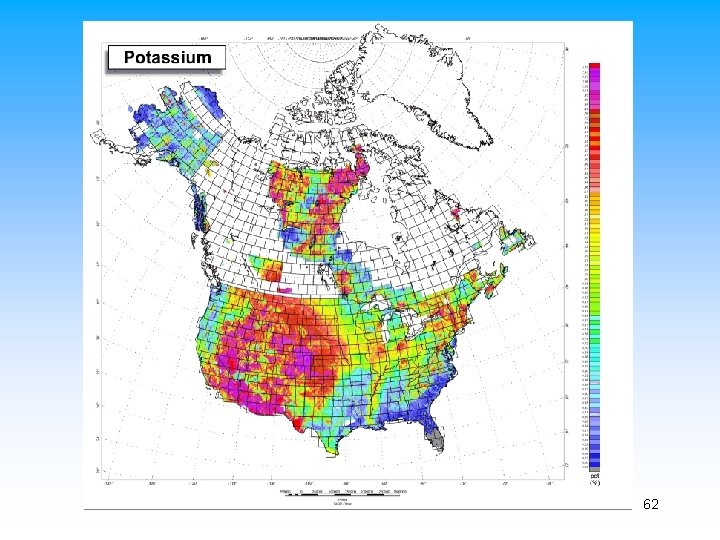
62
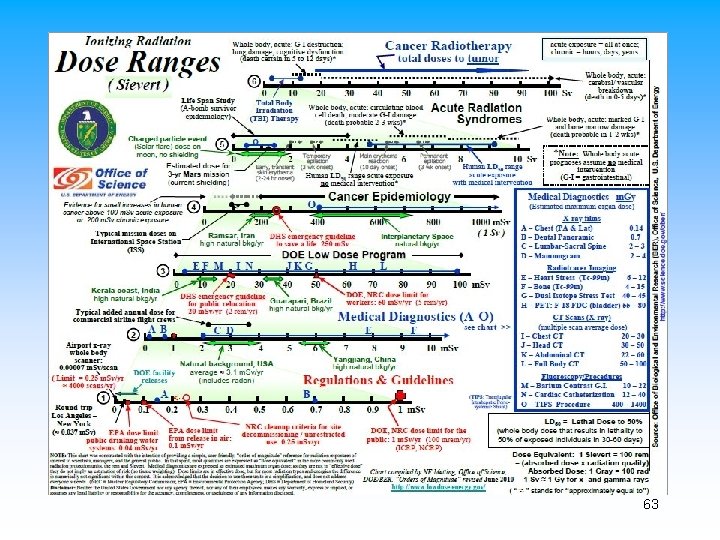
63
Gamma cameras bbc bitesize
Canadian bioinformatics workshop
Canadian bioinformatics workshop
Canadian bioinformatics workshop
Canadian bioinformatics workshop
Lesson 15 nuclear quest nuclear reactions
Fisión nuclear vs fision nuclear
Nuclear fission radiation
Types of radiation
What is nuclear radiation
Nuclear radiation
Synchrotron radiation workshop
Canadian evaluation society
Triple e bottom line
Canadian society of clinical perfusion
Team building training content
Team building workshop agenda
Ltpd抽樣表
Naas cns
Cns ischemic response
Histology of nervous system ppt
Composition of cns
Cns ischemic response
Mean arterial pressure
Cns ischemic response
Www.lispa.it cns
Ans and cns difference
Cns depressants ppt
Cns
Depresori cns
Cns international school
Cns poruchy
Grades of power cns
Dermatomes
Neutron capture
Sensory system
Cns ward
Cns
Image of central nervous system
Edrolo
Cns classification
Soma cns
Epineurium
Cholinergic drugs classification
Cns educar
Soma cns
Cns
Cnscp
Reeling gait
Depresori cns
Cns 15506
"the knowledge society" "the knowledge society" or tks
Team spirit becomes team infatuation
Team spirit becomes team infatuation
The white team cheers for the blue team, just like
Natural vegetation
Canadian registered safety professional designation
Forensic ballistics canada
Dimensions of globalisation
Canadian sheild facts
Marketing an introduction 6th canadian edition
Canadian forest food chain
Canadian engineering accreditation board
Canadian league against epilepsy