Transverse Spin and TMDs from SIDIS with Transversely
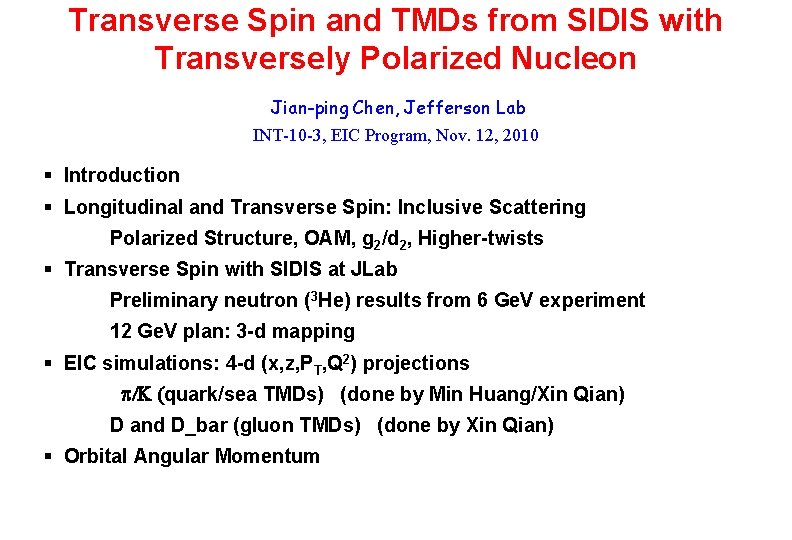
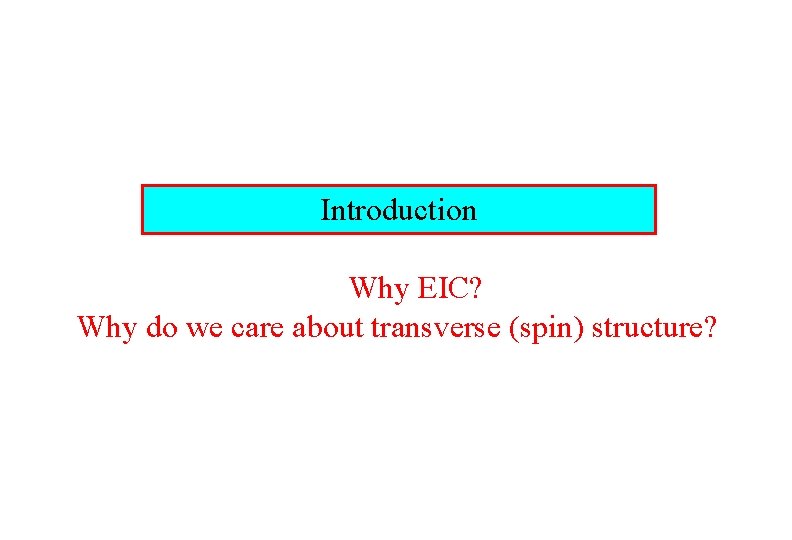
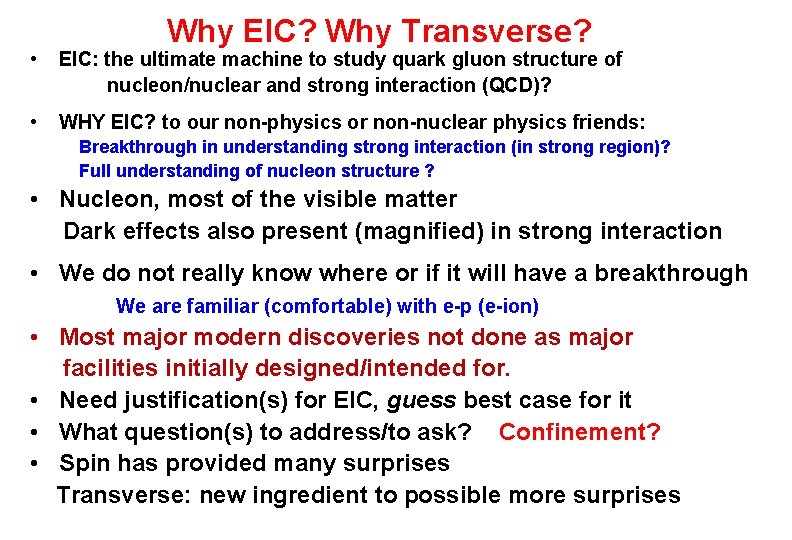
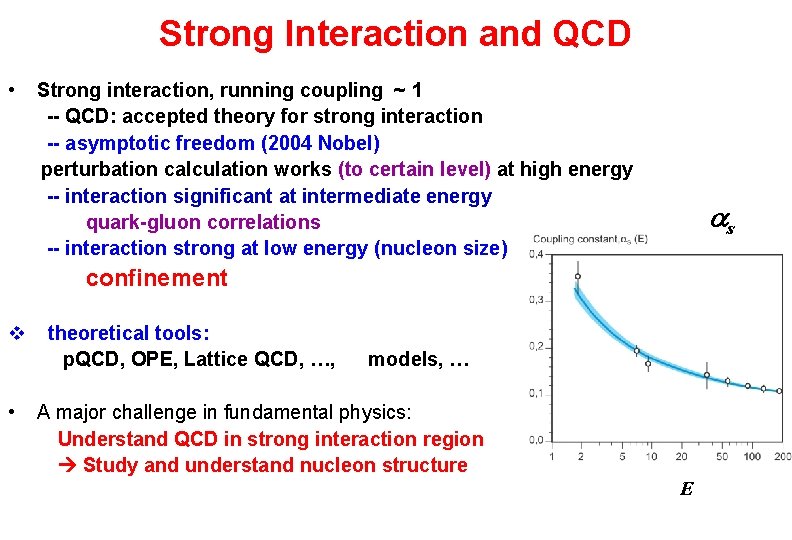
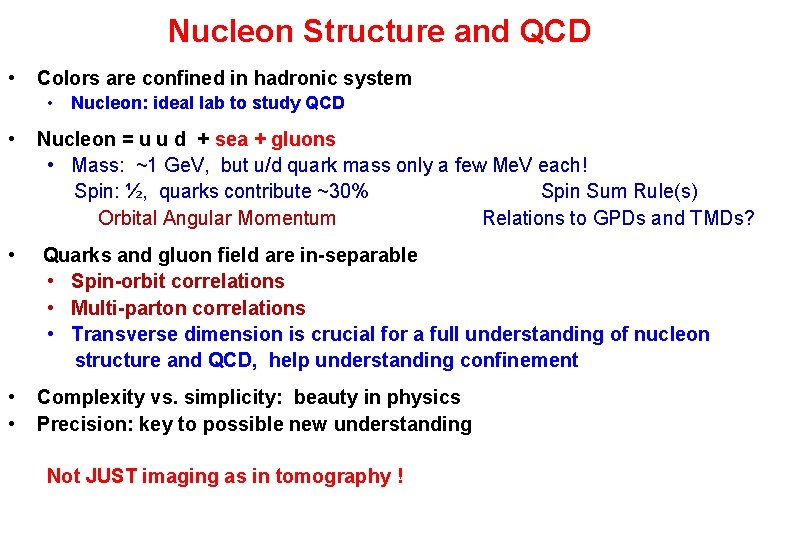
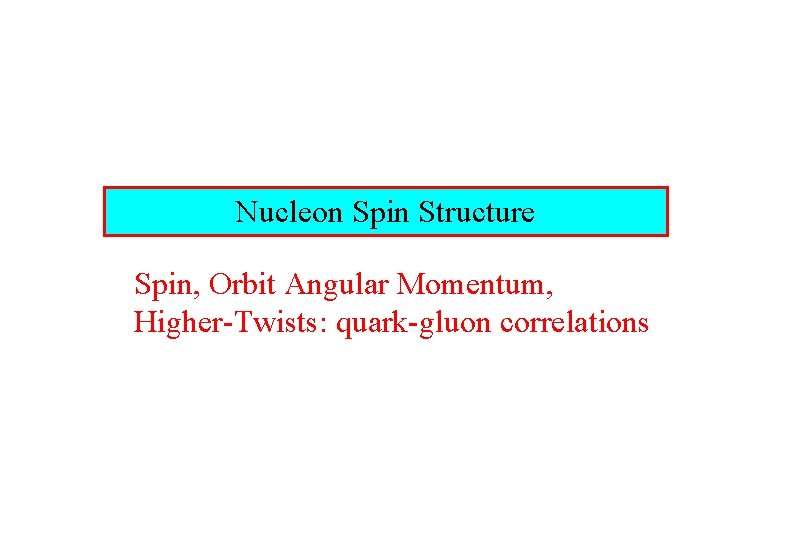
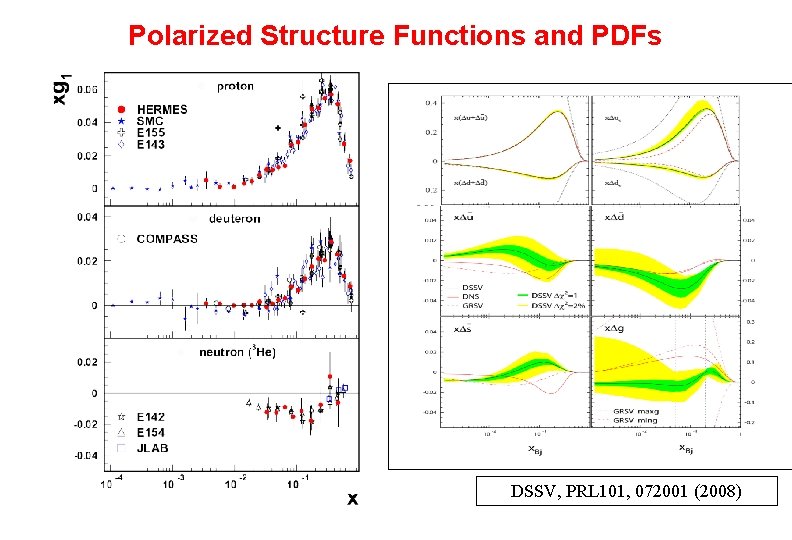
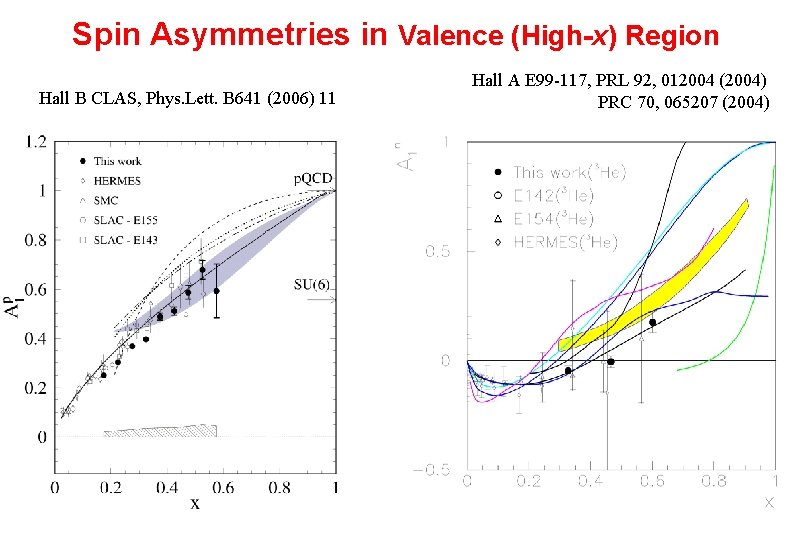
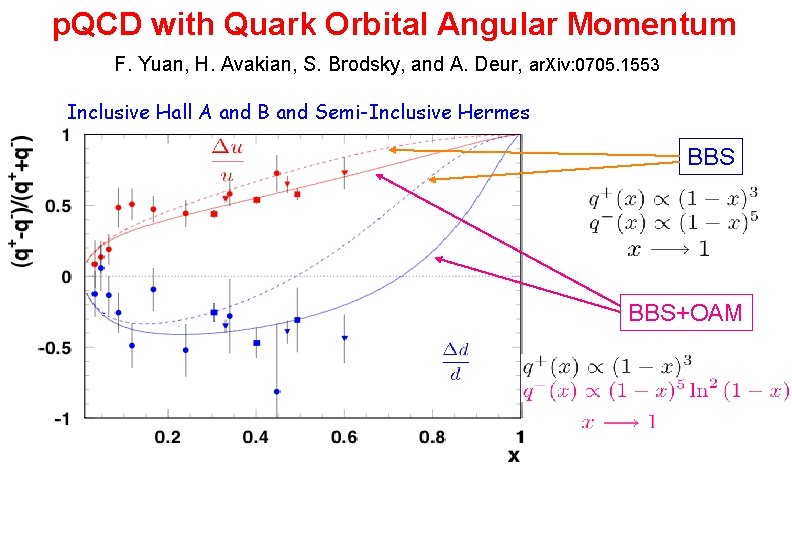
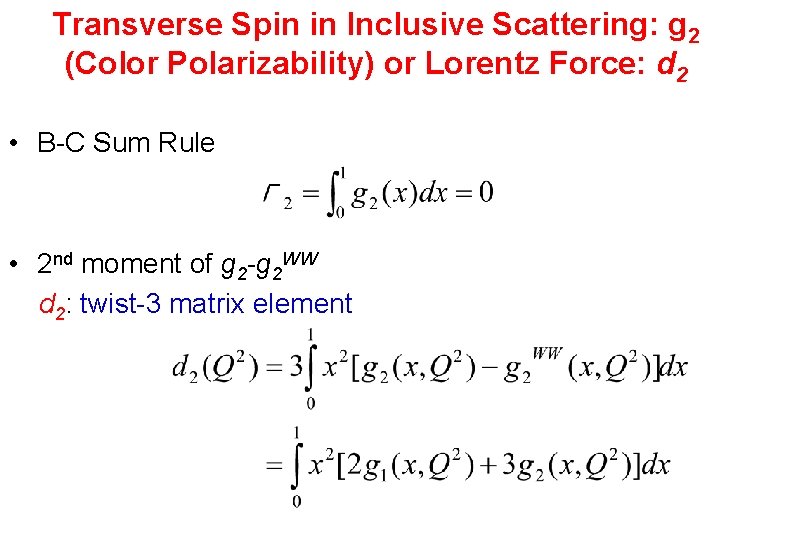
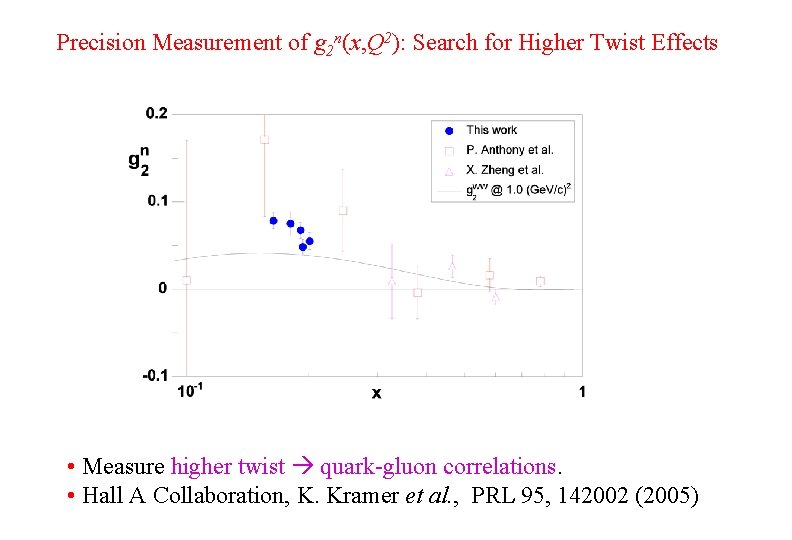
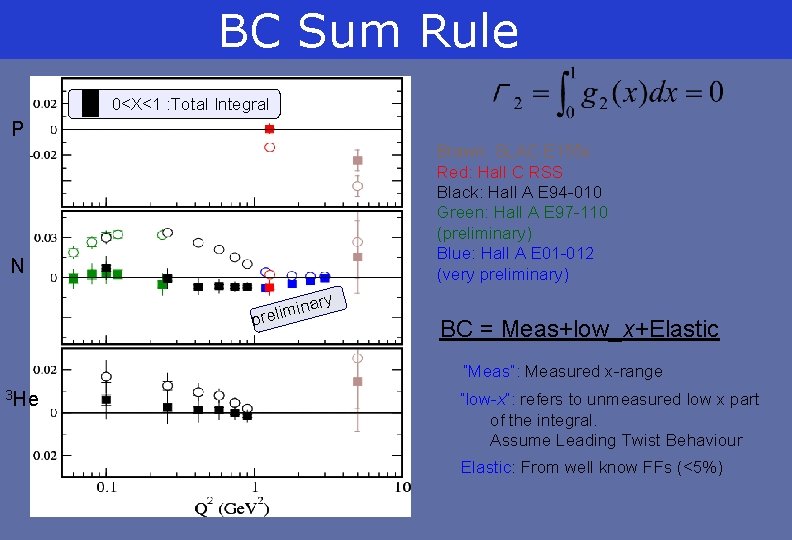
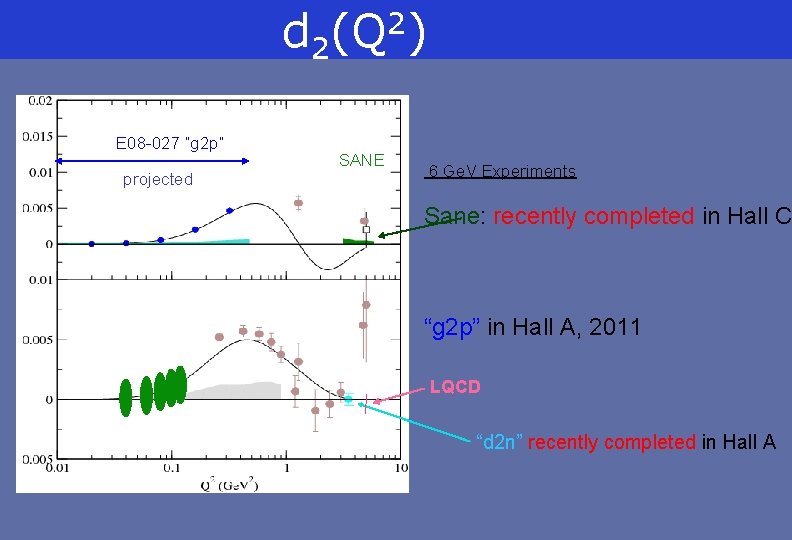
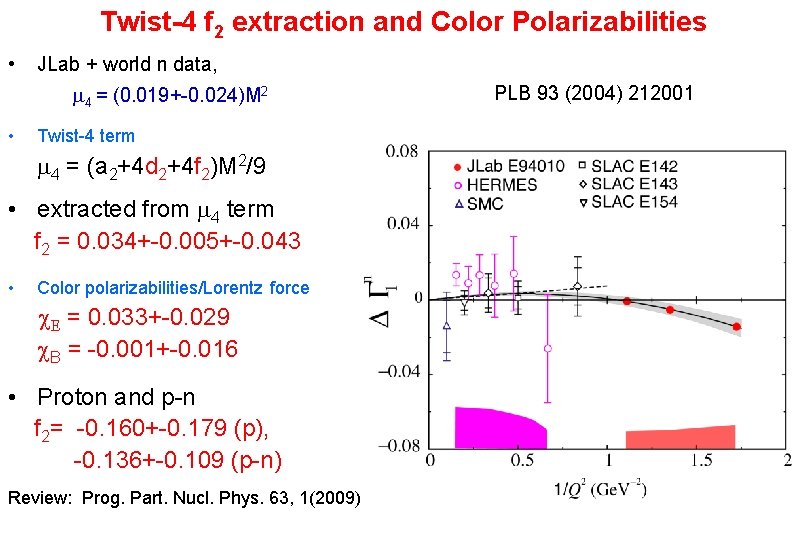
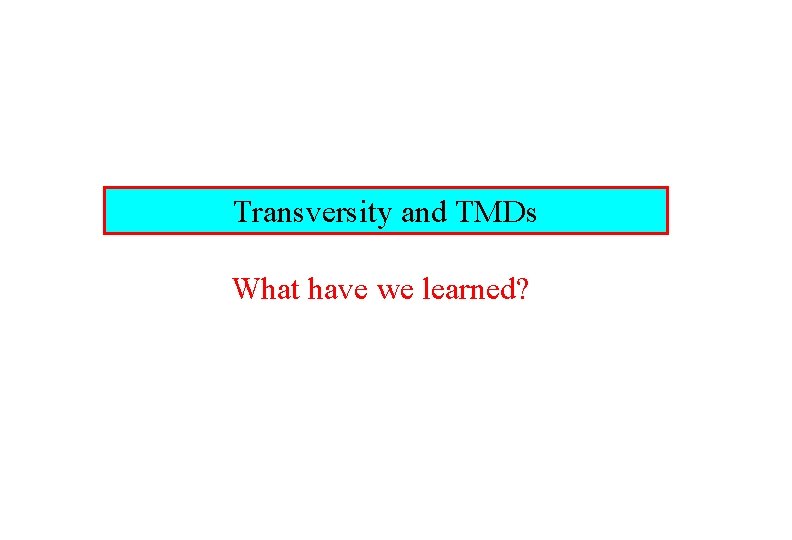
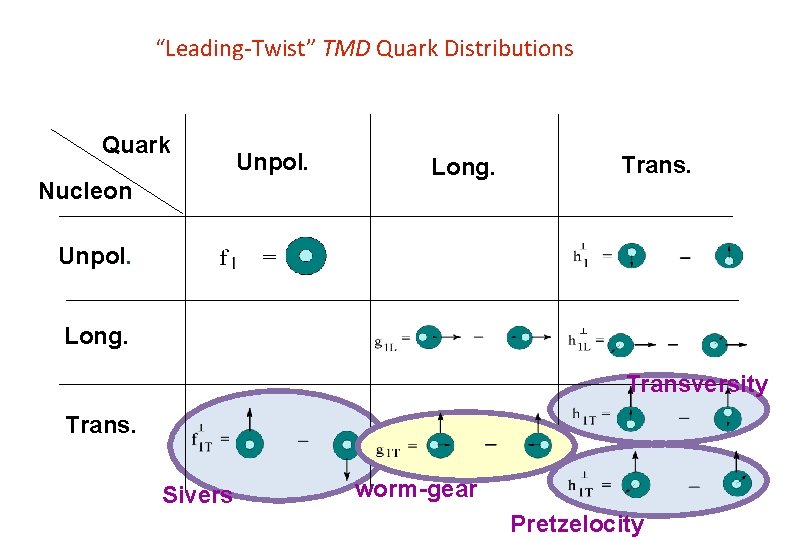
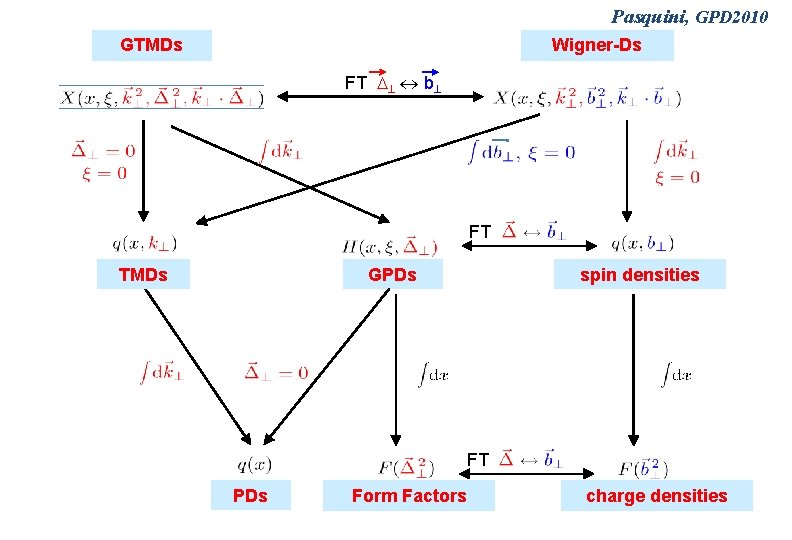
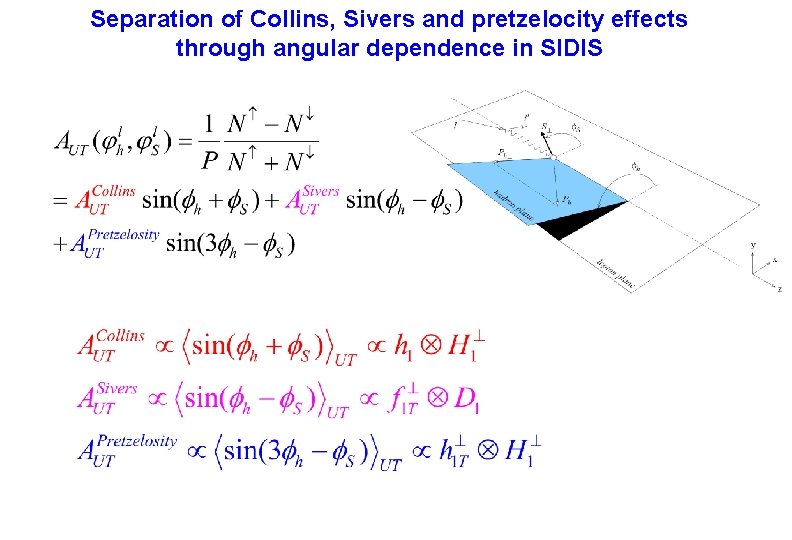
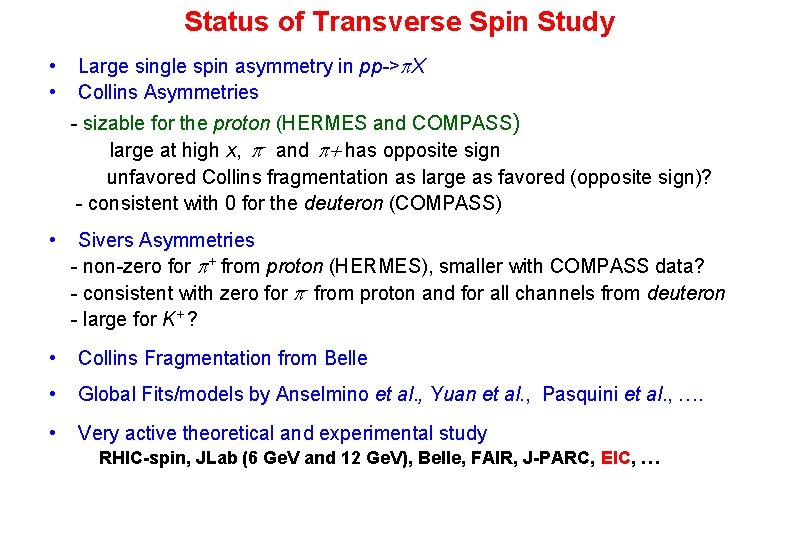
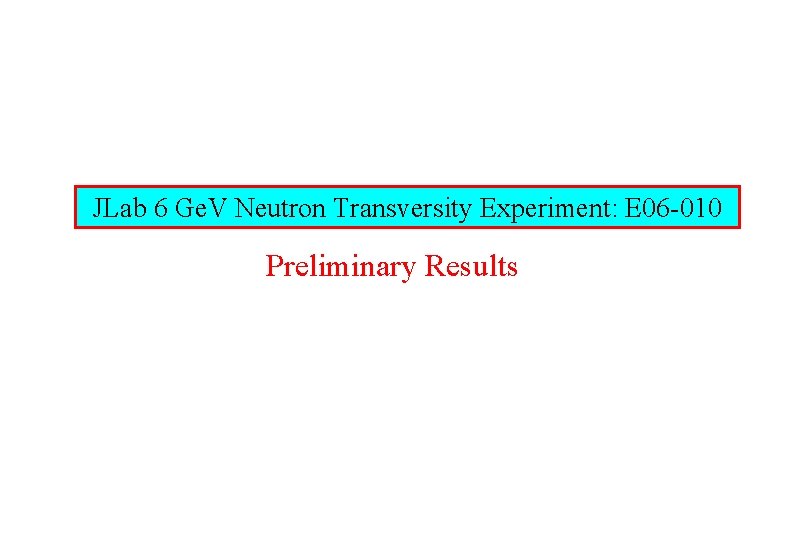
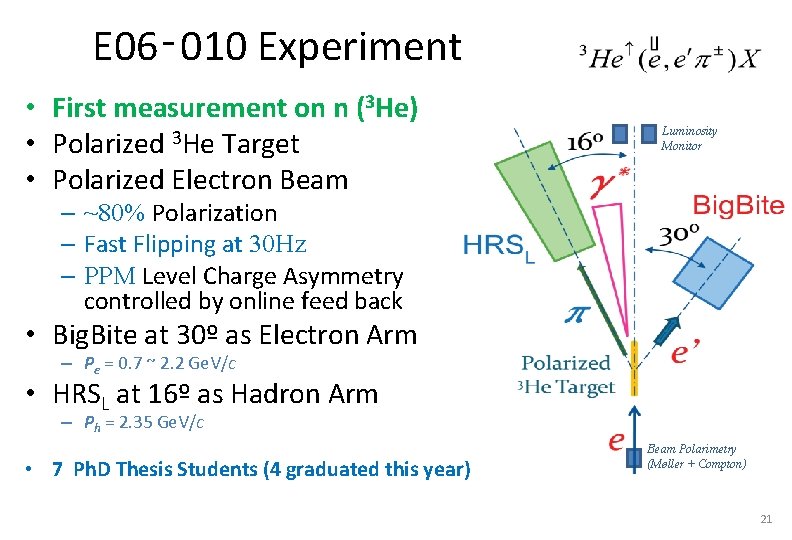
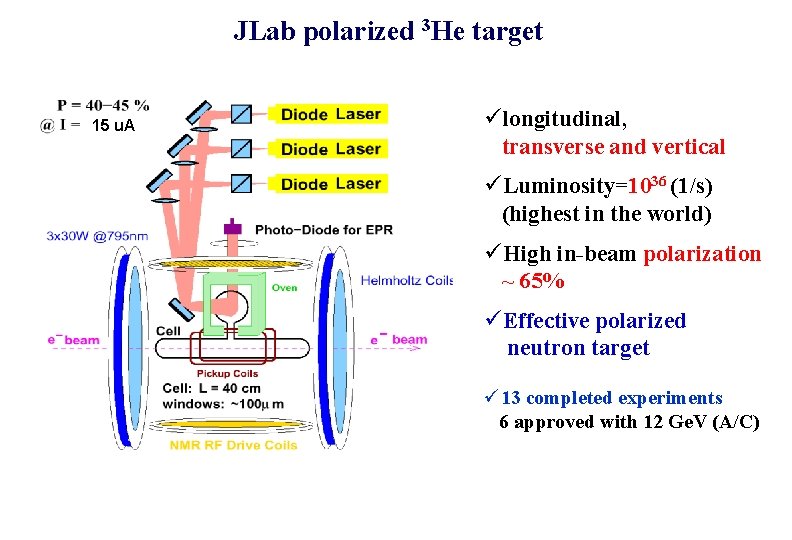
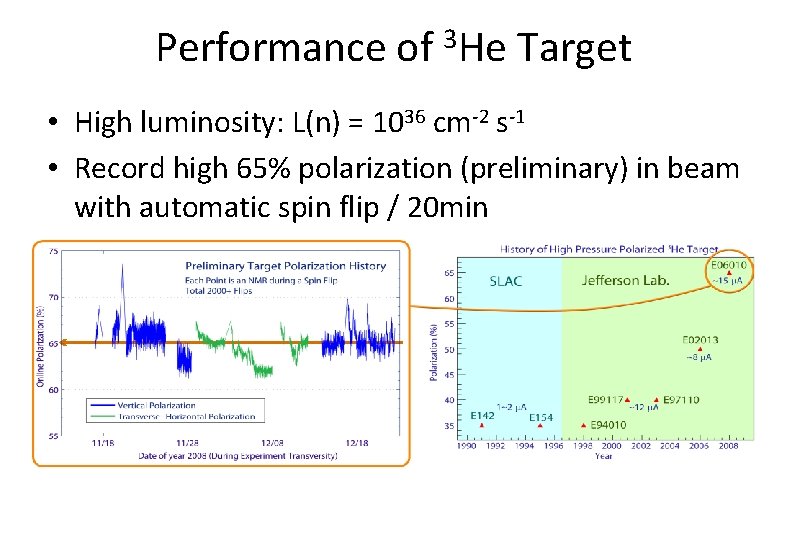
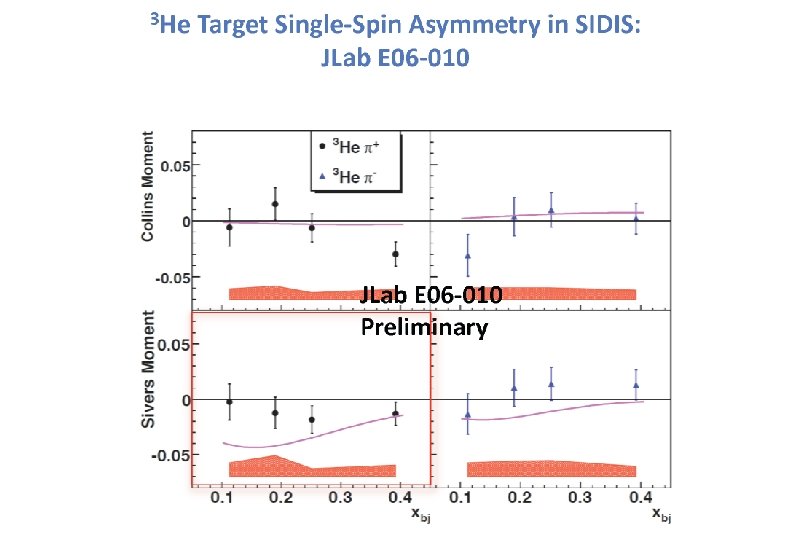
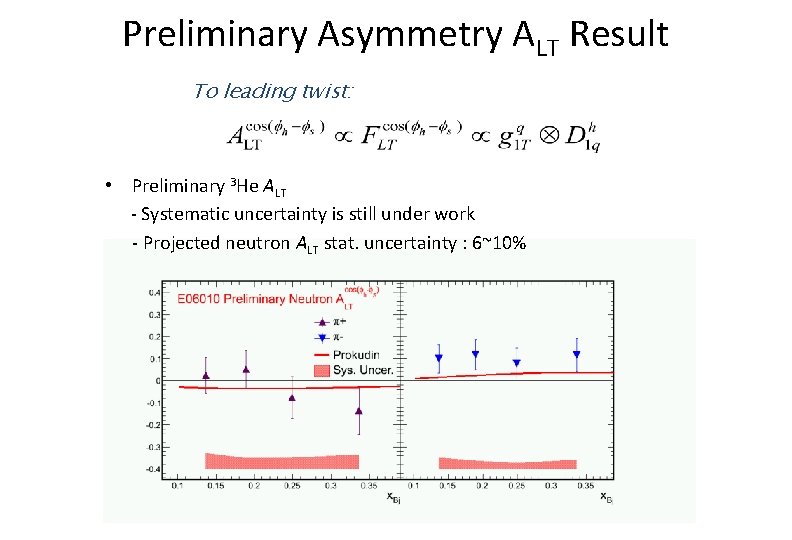
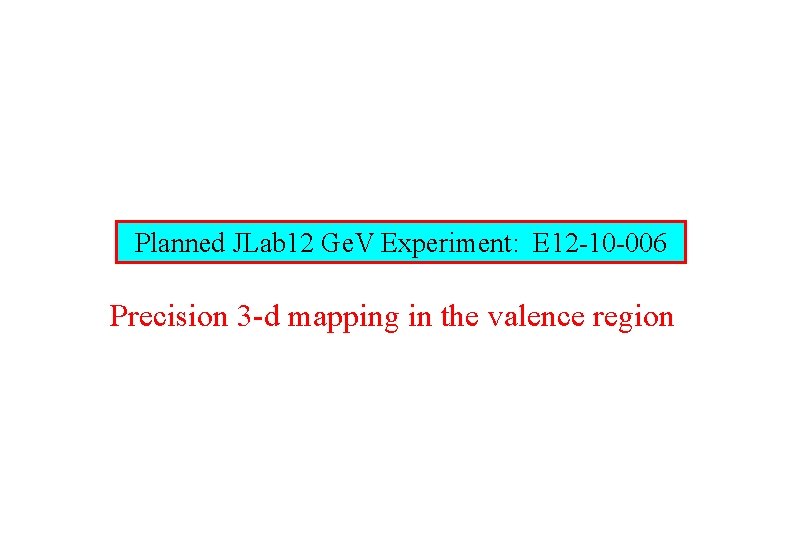
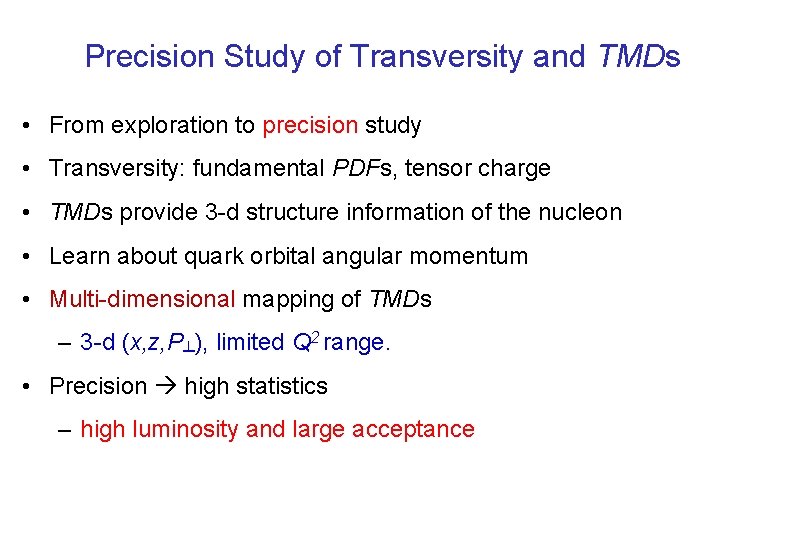
![Solenoid detector for SIDIS at 11 Ge. V Y[cm] Yoke Coil 3 He Target Solenoid detector for SIDIS at 11 Ge. V Y[cm] Yoke Coil 3 He Target](https://slidetodoc.com/presentation_image_h2/f2e985179333e1a0b1e4396482423626/image-28.jpg)
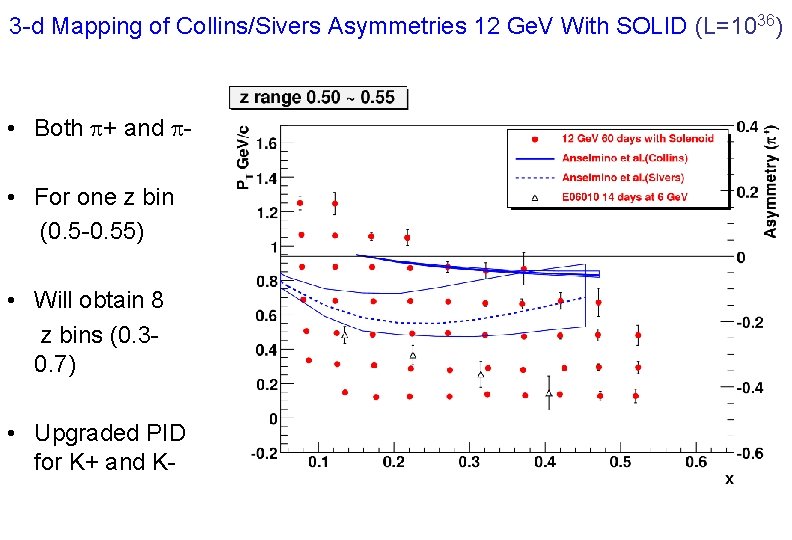
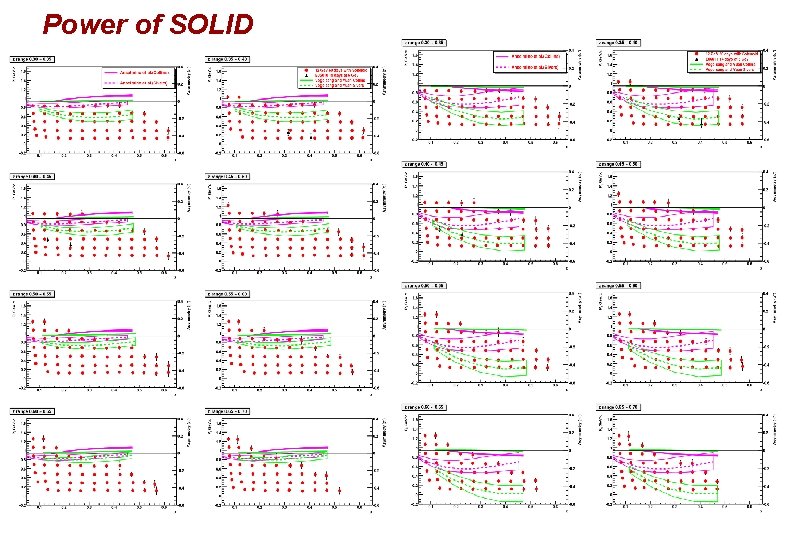
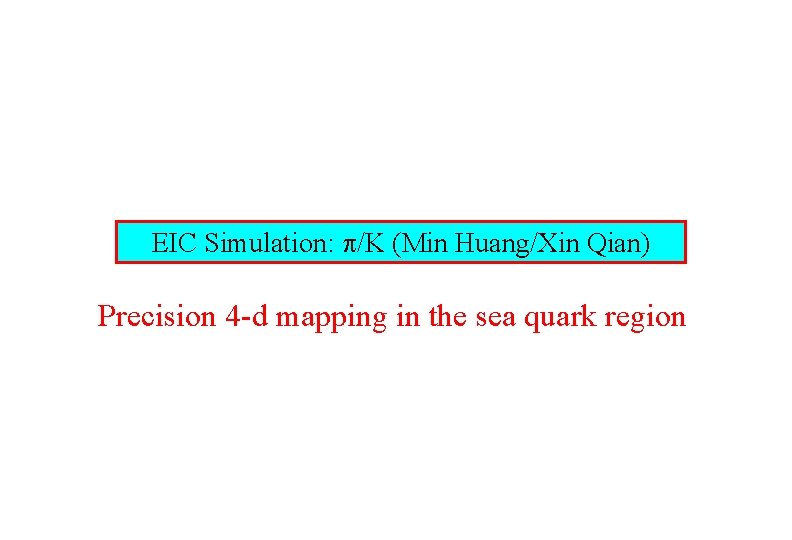
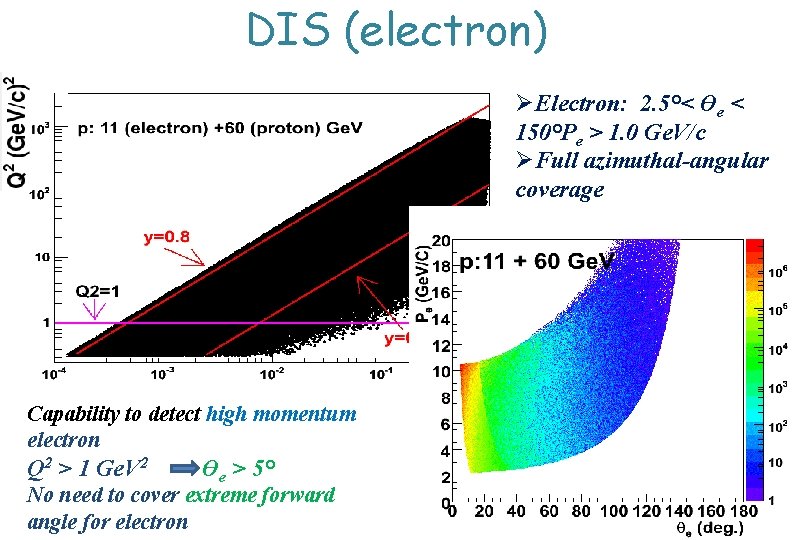
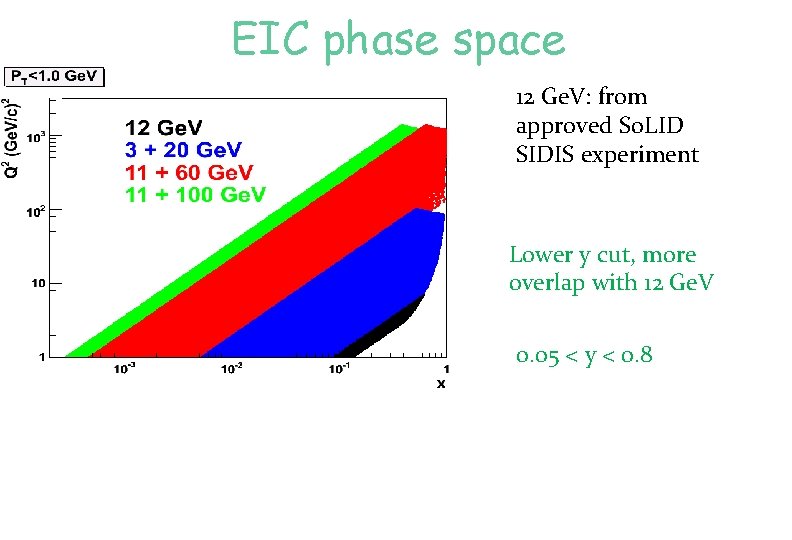
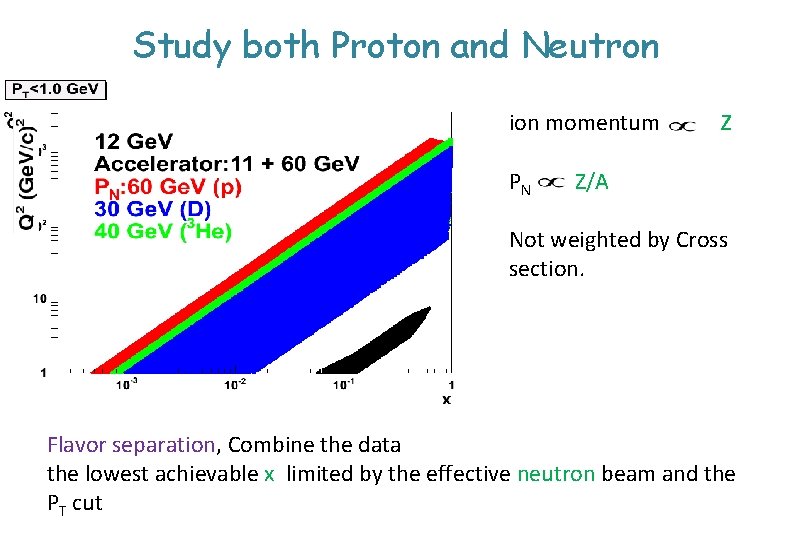
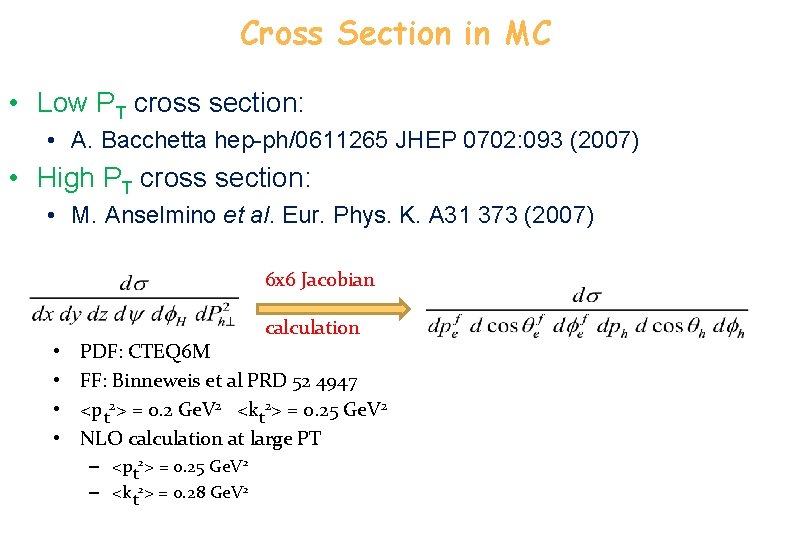
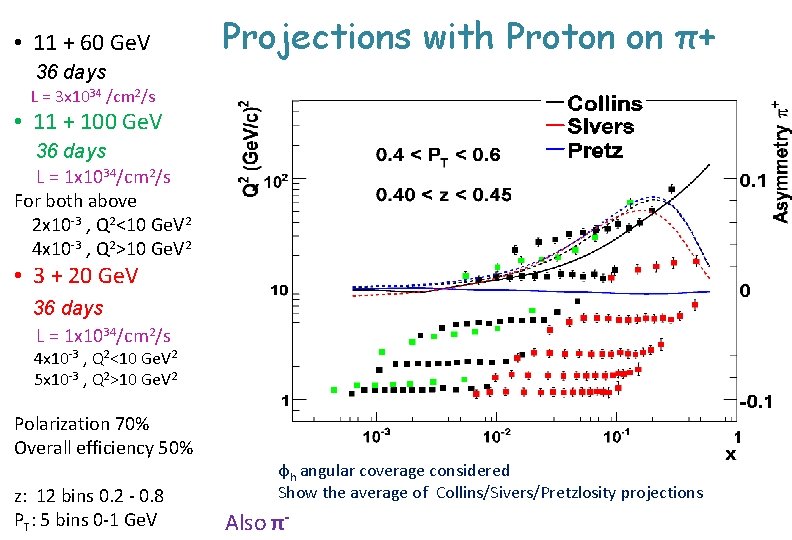
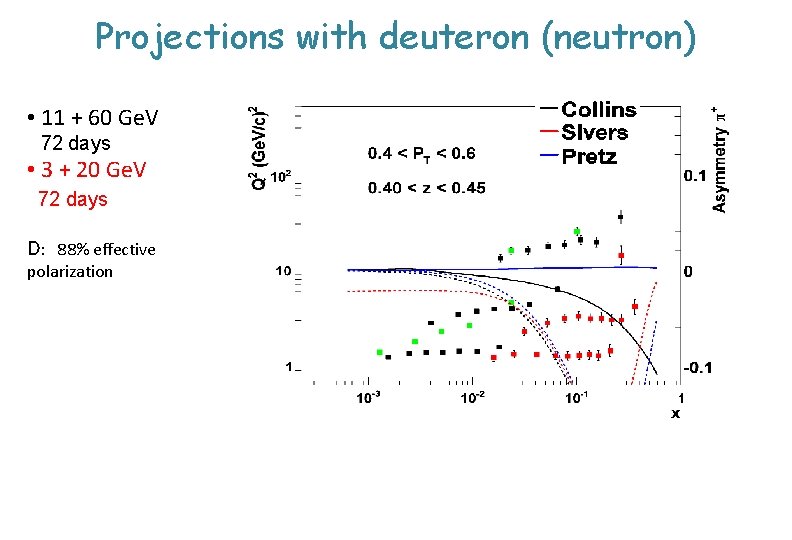
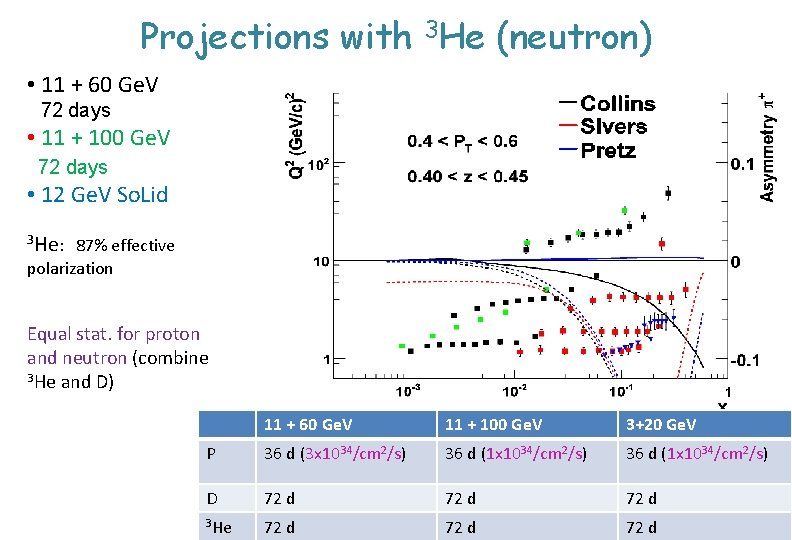
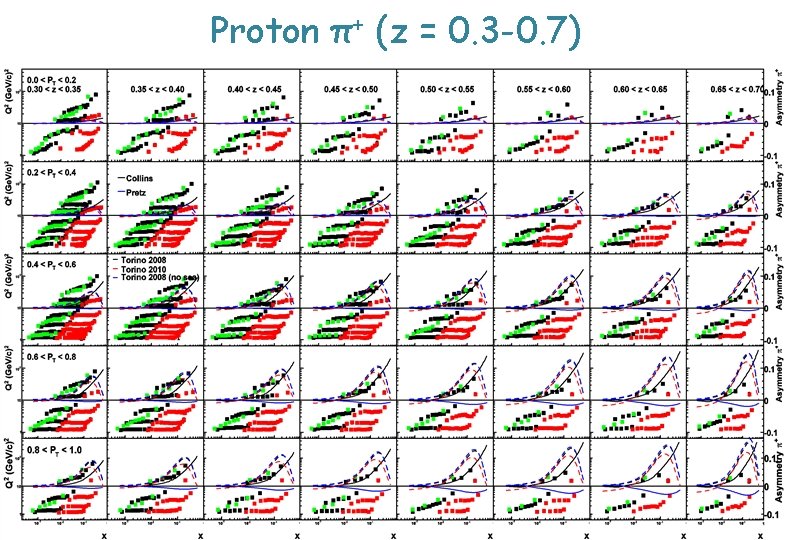
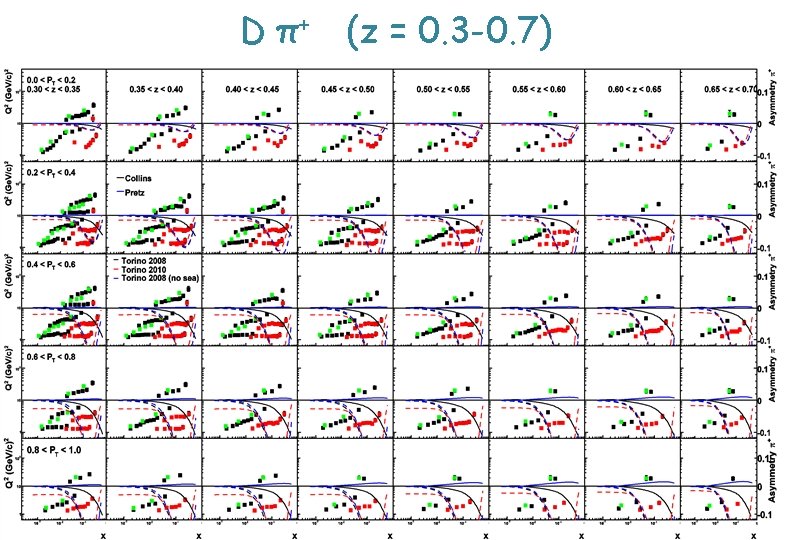
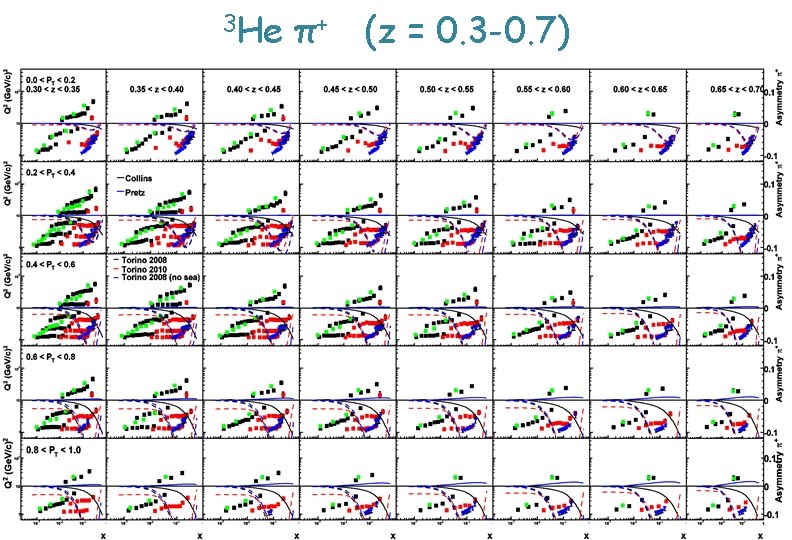
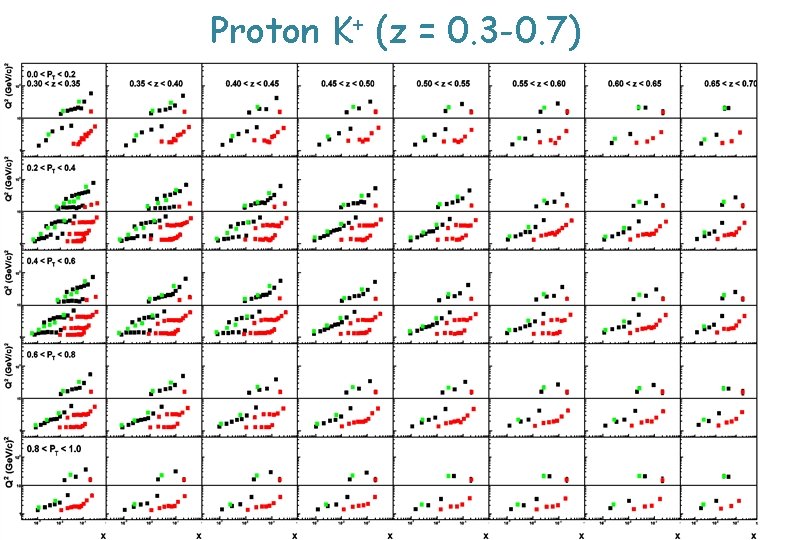
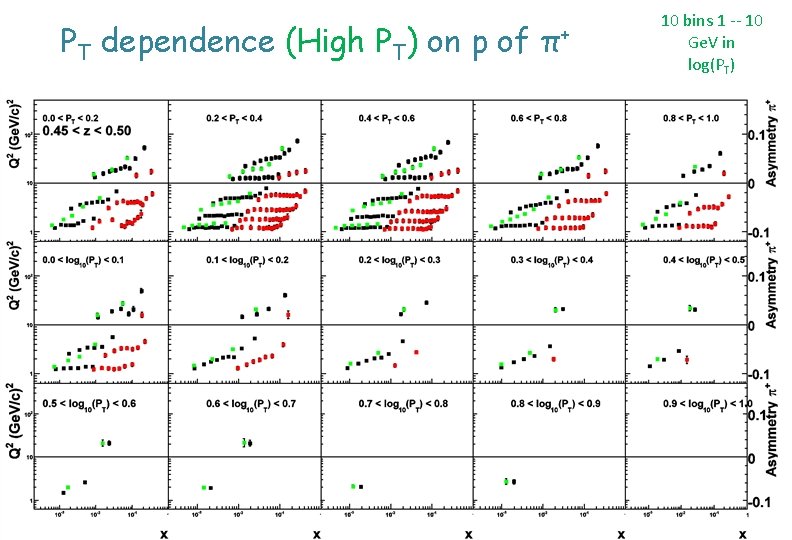
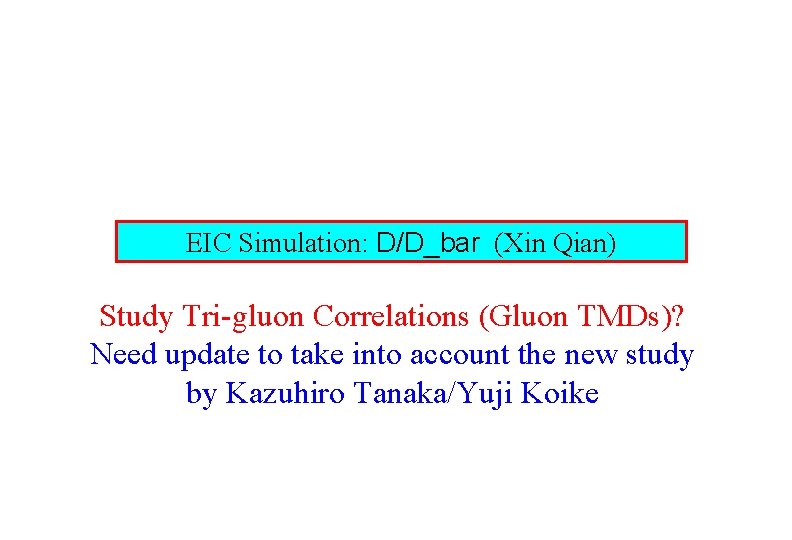
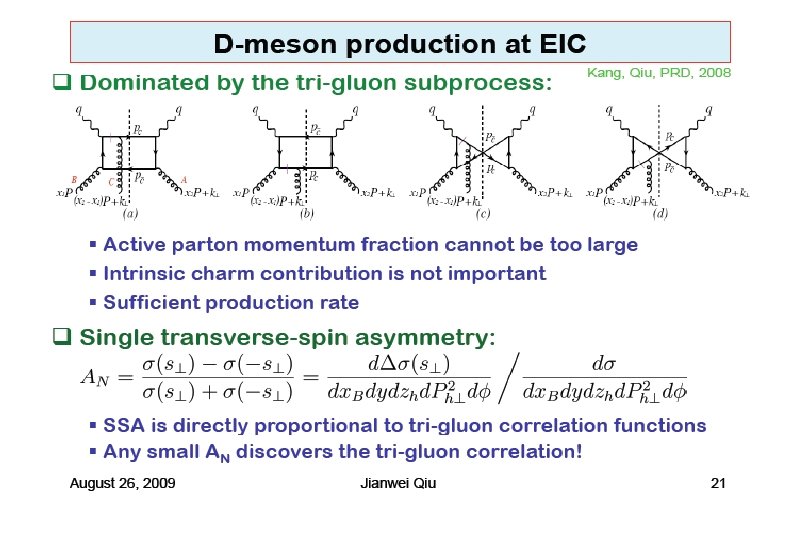
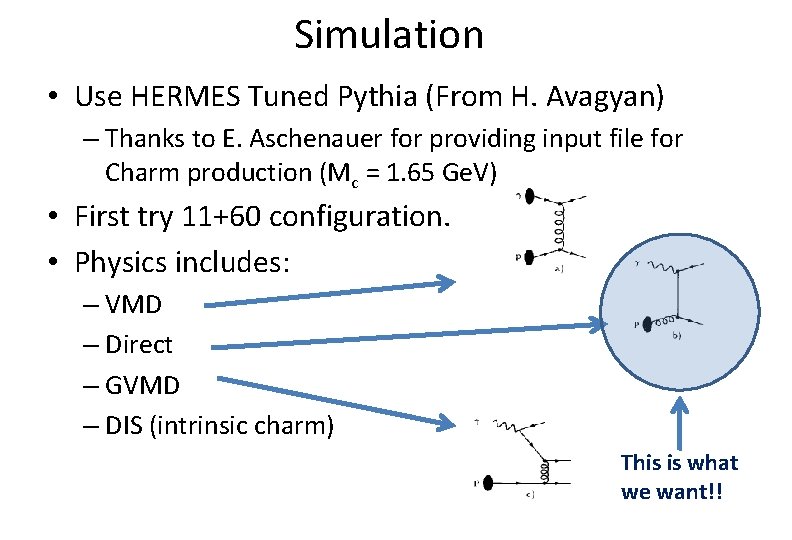
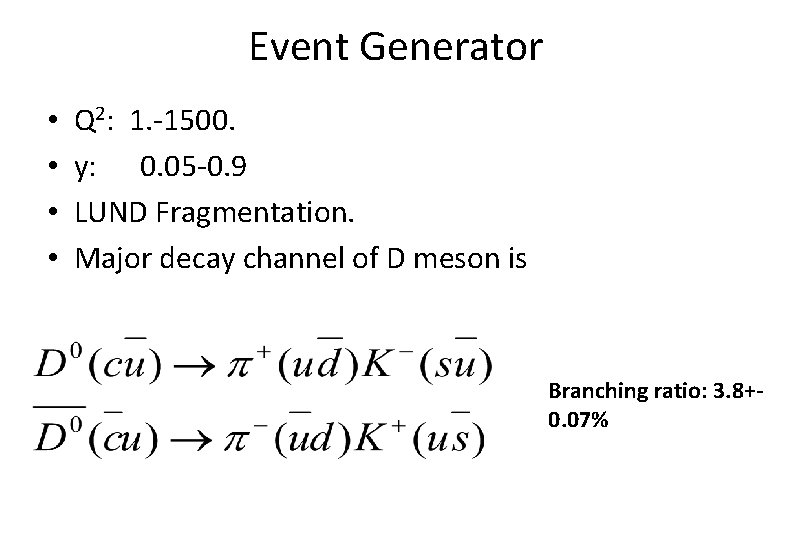
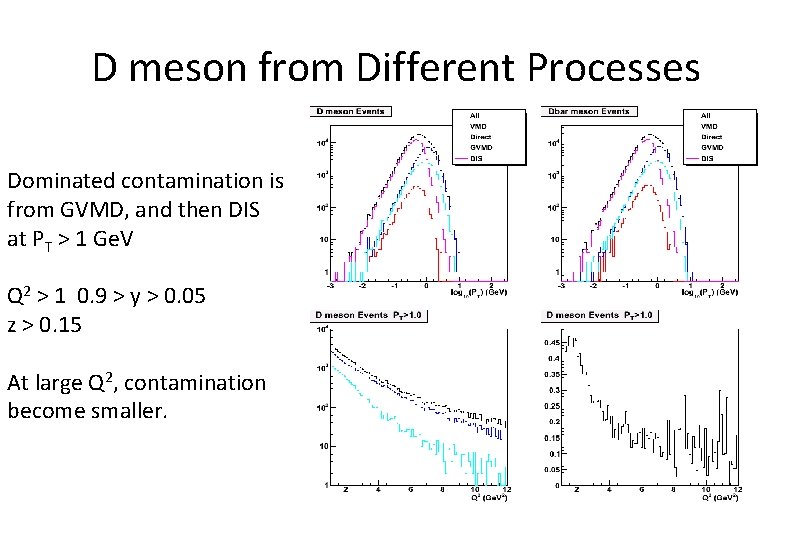
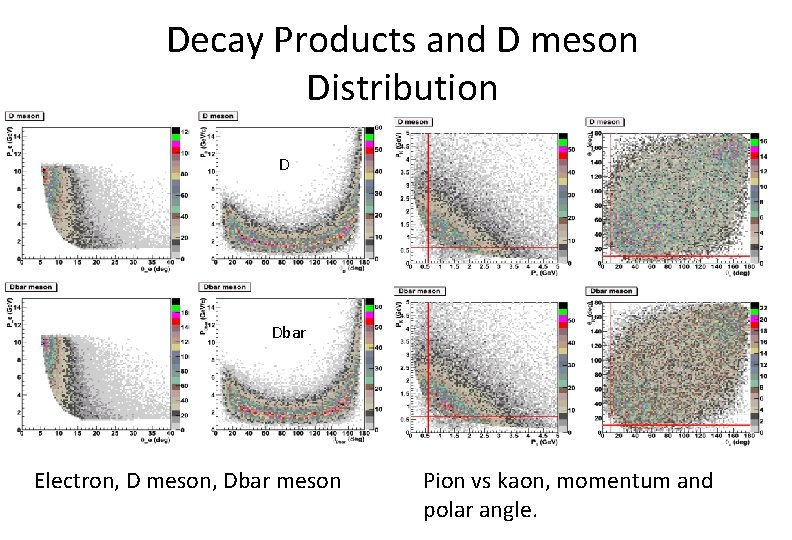
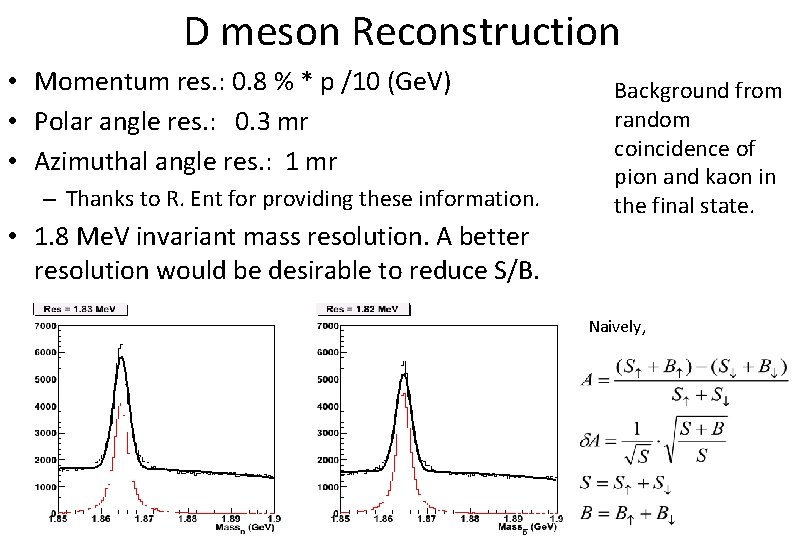
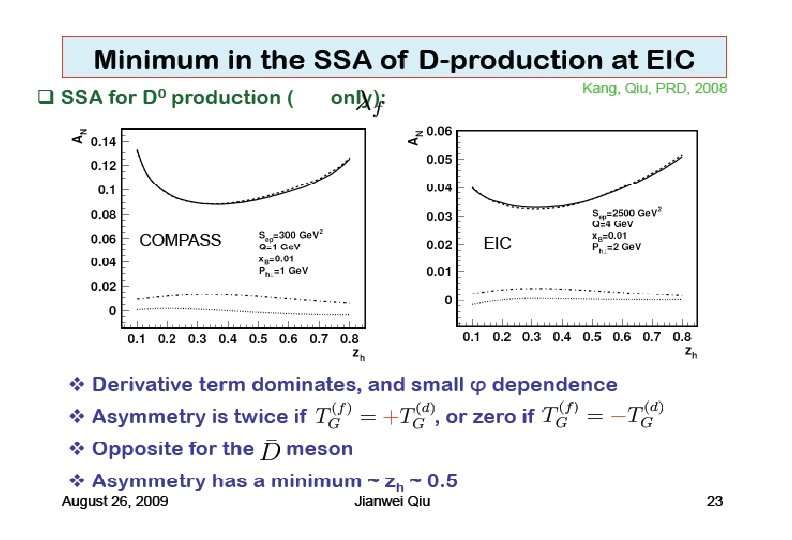
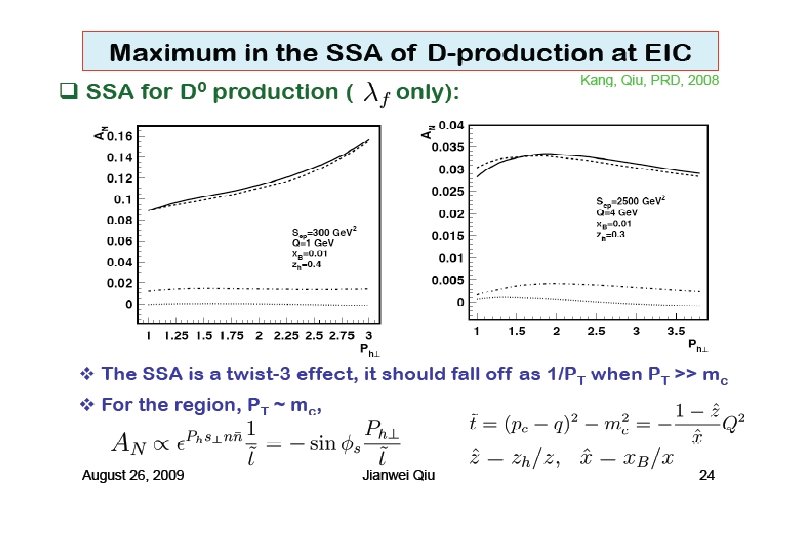
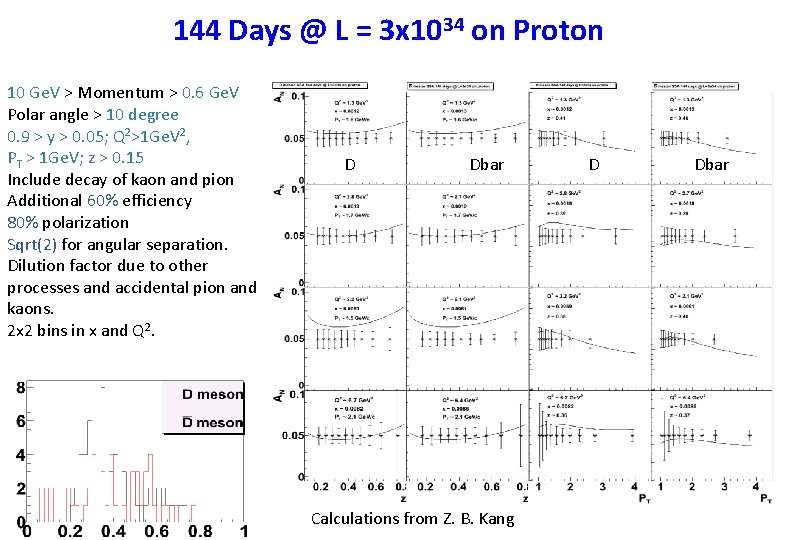
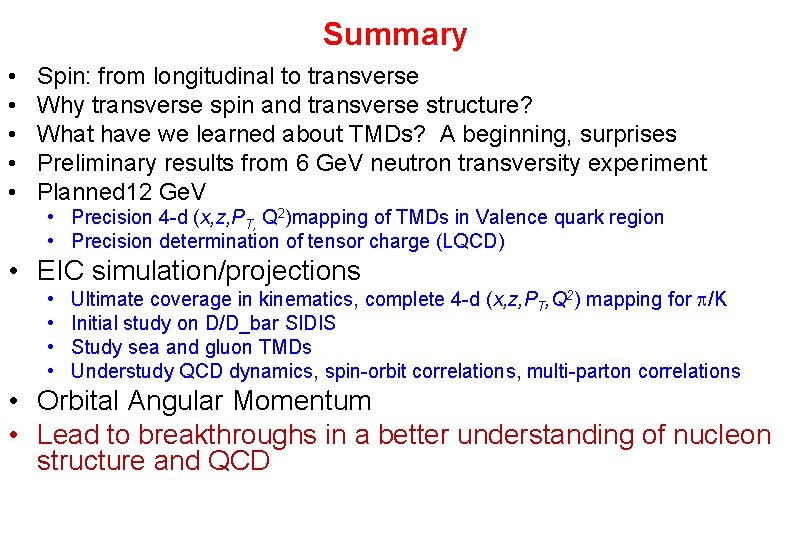
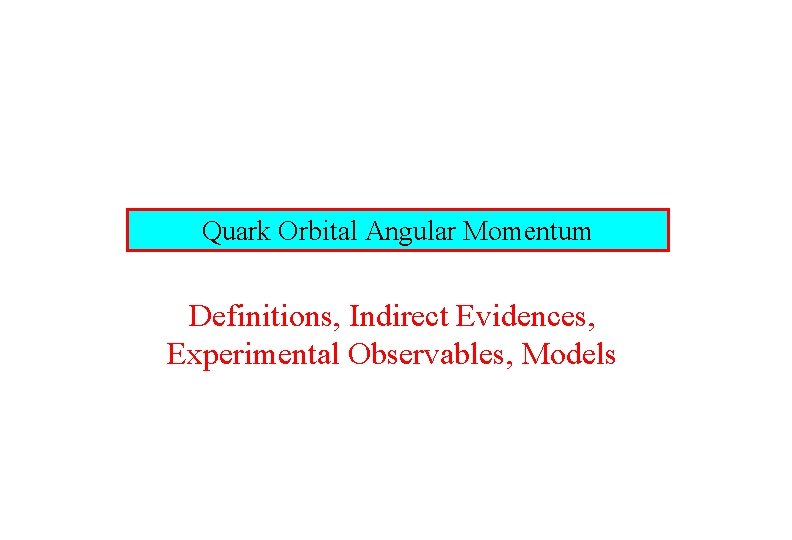
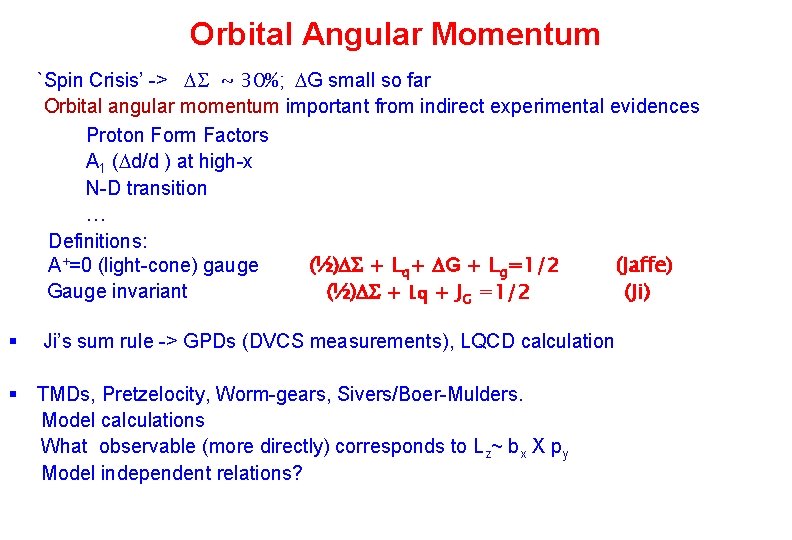
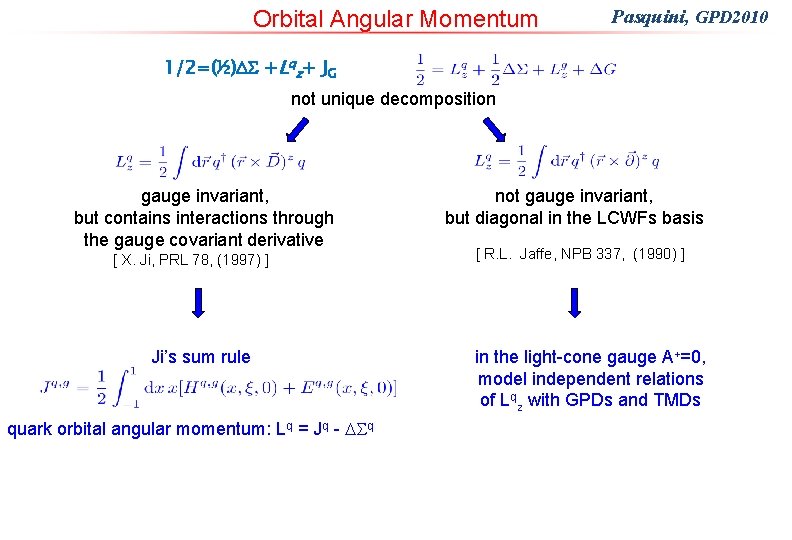
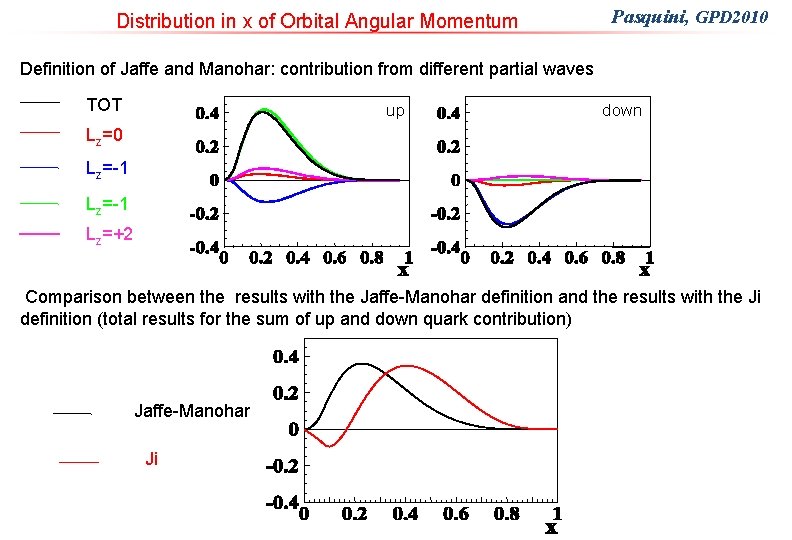
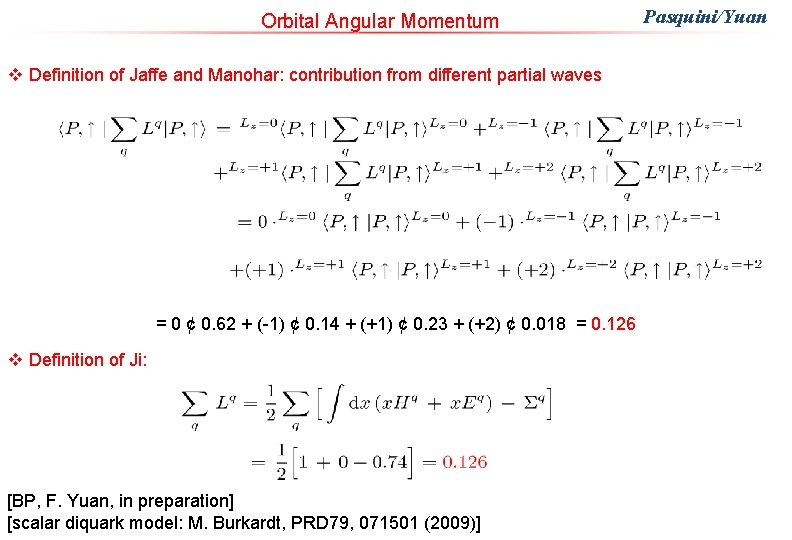
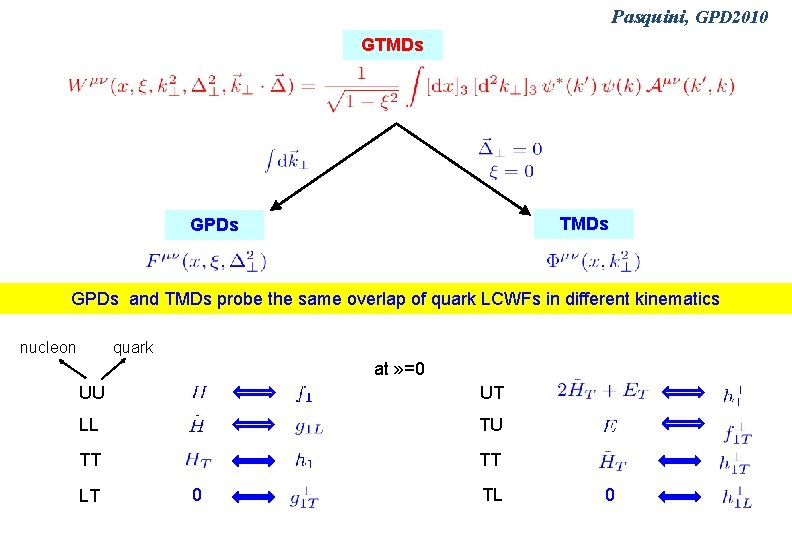
- Slides: 60
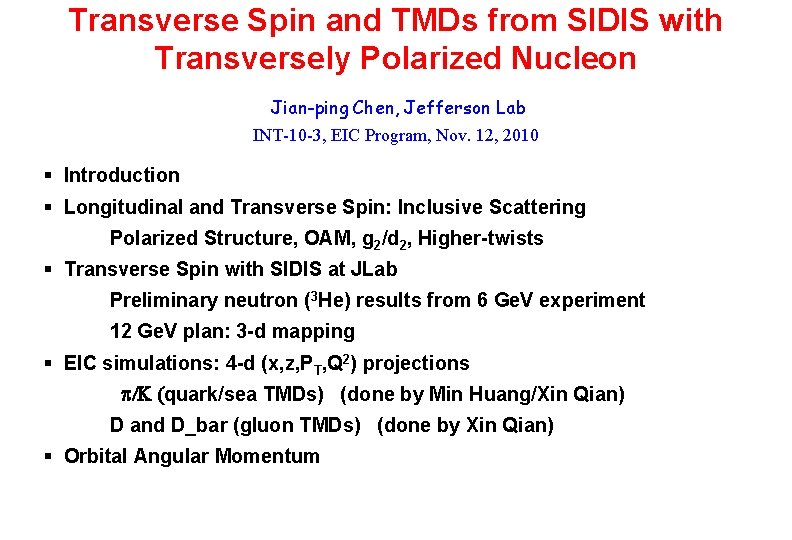
Transverse Spin and TMDs from SIDIS with Transversely Polarized Nucleon Jian-ping Chen, Jefferson Lab INT-10 -3, EIC Program, Nov. 12, 2010 § Introduction § Longitudinal and Transverse Spin: Inclusive Scattering Polarized Structure, OAM, g 2/d 2, Higher-twists § Transverse Spin with SIDIS at JLab Preliminary neutron (3 He) results from 6 Ge. V experiment 12 Ge. V plan: 3 -d mapping § EIC simulations: 4 -d (x, z, PT, Q 2) projections p/K (quark/sea TMDs) (done by Min Huang/Xin Qian) D and D_bar (gluon TMDs) (done by Xin Qian) § Orbital Angular Momentum
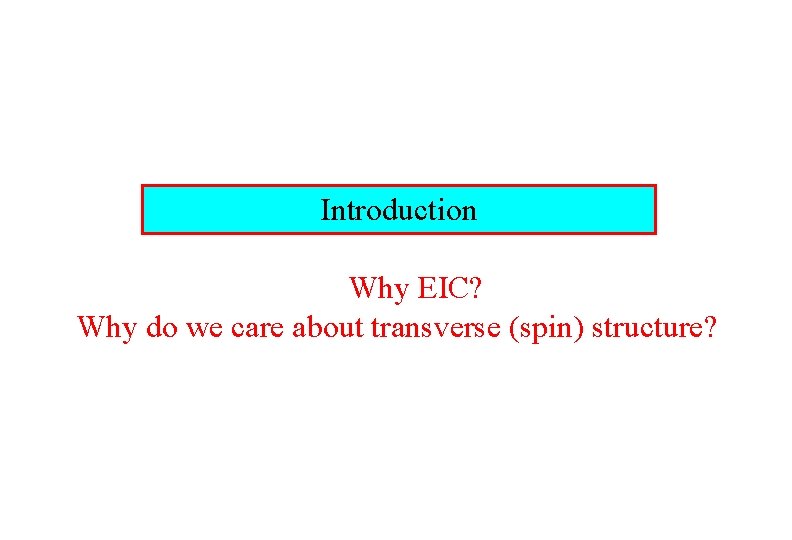
Introduction Why EIC? Why do we care about transverse (spin) structure?
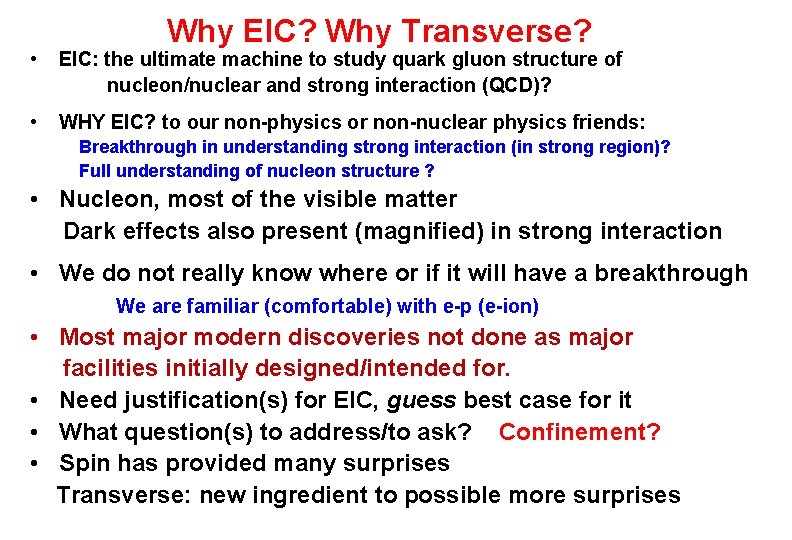
Why EIC? Why Transverse? • EIC: the ultimate machine to study quark gluon structure of nucleon/nuclear and strong interaction (QCD)? • WHY EIC? to our non-physics or non-nuclear physics friends: Breakthrough in understanding strong interaction (in strong region)? Full understanding of nucleon structure ? • Nucleon, most of the visible matter Dark effects also present (magnified) in strong interaction • We do not really know where or if it will have a breakthrough We are familiar (comfortable) with e-p (e-ion) • Most major modern discoveries not done as major facilities initially designed/intended for. • Need justification(s) for EIC, guess best case for it • What question(s) to address/to ask? Confinement? • Spin has provided many surprises Transverse: new ingredient to possible more surprises
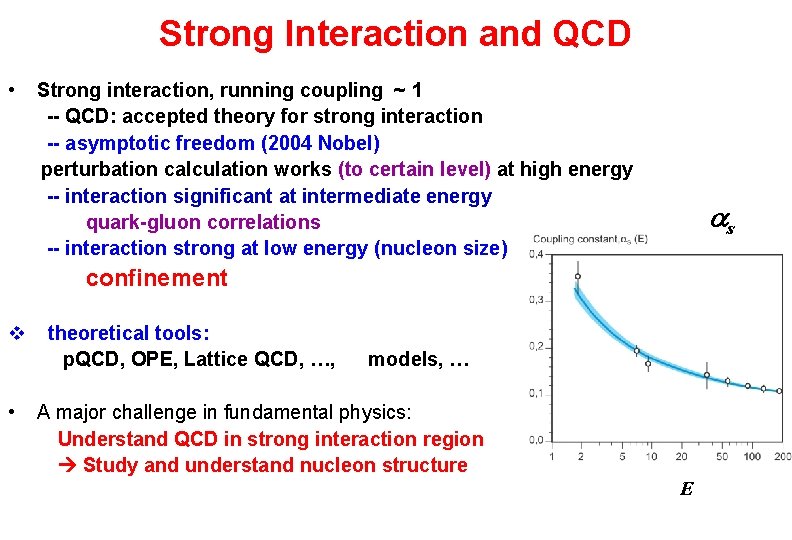
Strong Interaction and QCD • Strong interaction, running coupling ~ 1 -- QCD: accepted theory for strong interaction -- asymptotic freedom (2004 Nobel) perturbation calculation works (to certain level) at high energy -- interaction significant at intermediate energy quark-gluon correlations -- interaction strong at low energy (nucleon size) as confinement v • theoretical tools: p. QCD, OPE, Lattice QCD, …, models, … A major challenge in fundamental physics: Understand QCD in strong interaction region Study and understand nucleon structure E
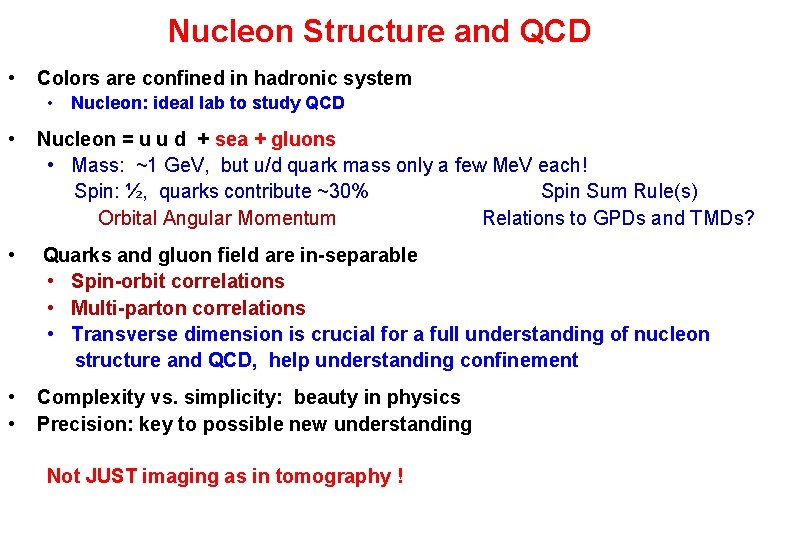
Nucleon Structure and QCD • Colors are confined in hadronic system • Nucleon: ideal lab to study QCD • Nucleon = u u d + sea + gluons • Mass: ~1 Ge. V, but u/d quark mass only a few Me. V each! Spin: ½, quarks contribute ~30% Spin Sum Rule(s) Orbital Angular Momentum Relations to GPDs and TMDs? • Quarks and gluon field are in-separable • Spin-orbit correlations • Multi-parton correlations • Transverse dimension is crucial for a full understanding of nucleon structure and QCD, help understanding confinement • • Complexity vs. simplicity: beauty in physics Precision: key to possible new understanding Not JUST imaging as in tomography !
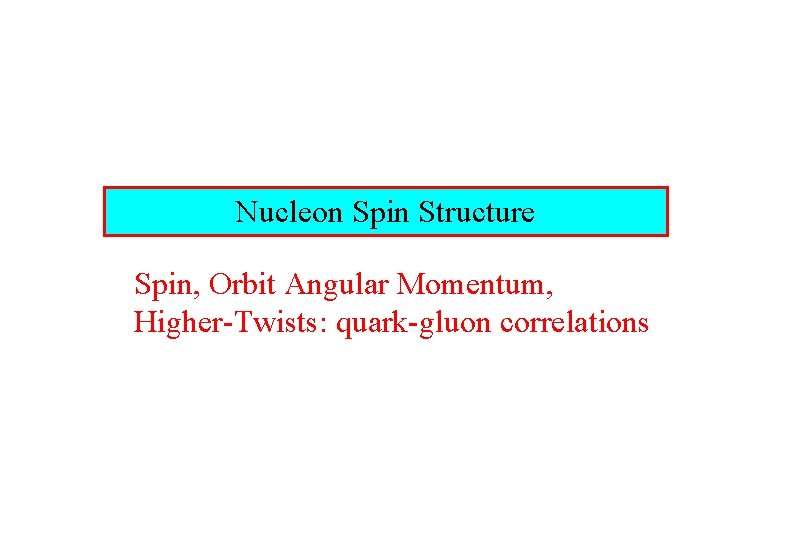
Nucleon Spin Structure Spin, Orbit Angular Momentum, Higher-Twists: quark-gluon correlations
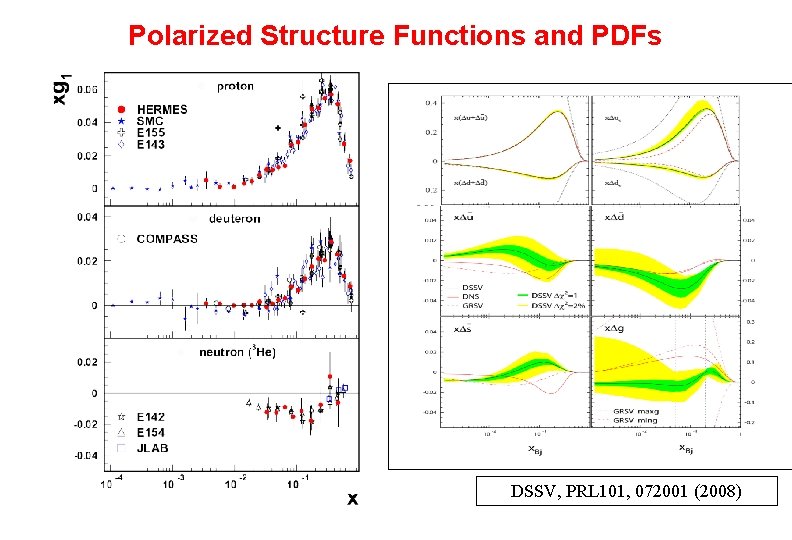
Polarized Structure Functions and PDFs DSSV, PRL 101, 072001 (2008)
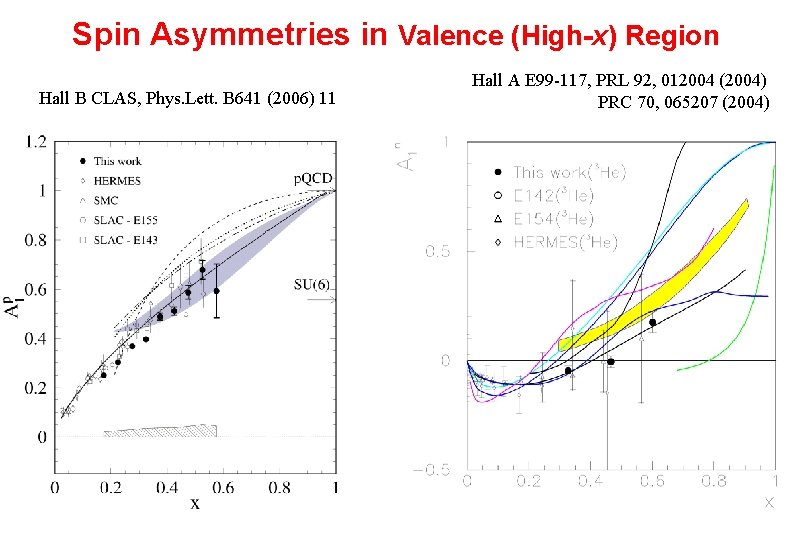
Spin Asymmetries in Valence (High-x) Region Hall B CLAS, Phys. Lett. B 641 (2006) 11 Hall A E 99 -117, PRL 92, 012004 (2004) PRC 70, 065207 (2004)
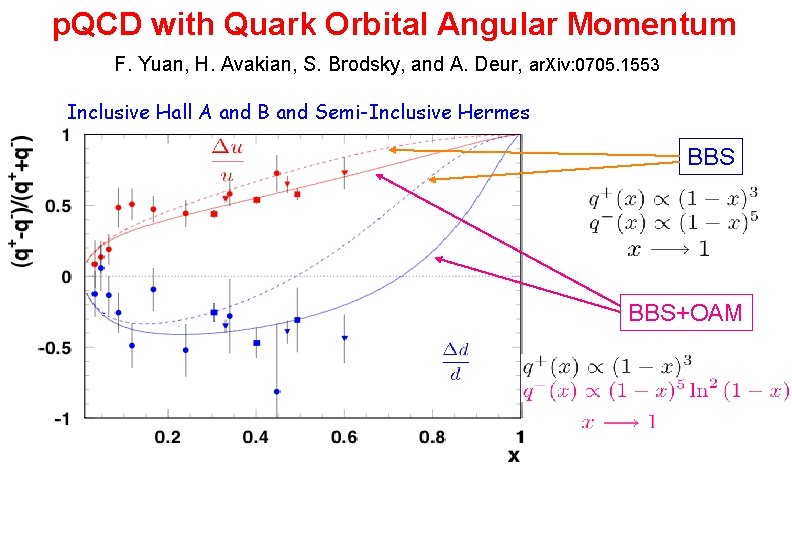
p. QCD with Quark Orbital Angular Momentum F. Yuan, H. Avakian, S. Brodsky, and A. Deur, ar. Xiv: 0705. 1553 Inclusive Hall A and B and Semi-Inclusive Hermes BBS+OAM
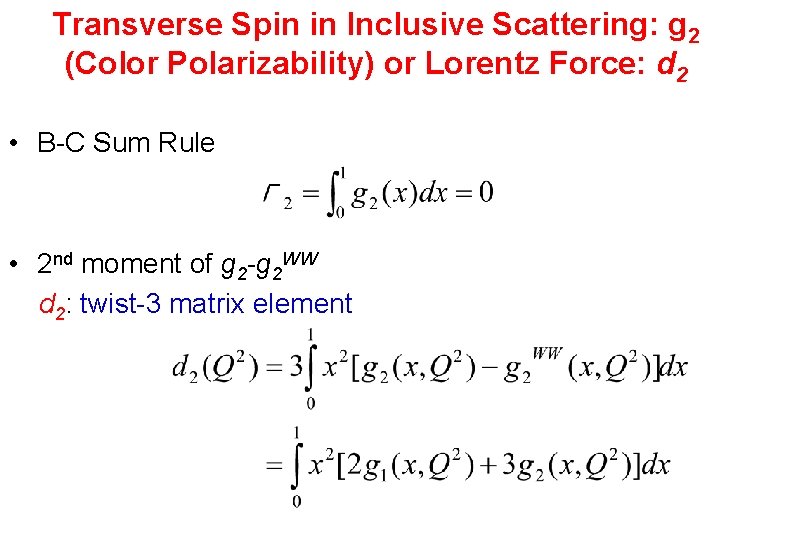
Transverse Spin in Inclusive Scattering: g 2 (Color Polarizability) or Lorentz Force: d 2 • B-C Sum Rule • 2 nd moment of g 2 -g 2 WW d 2: twist-3 matrix element
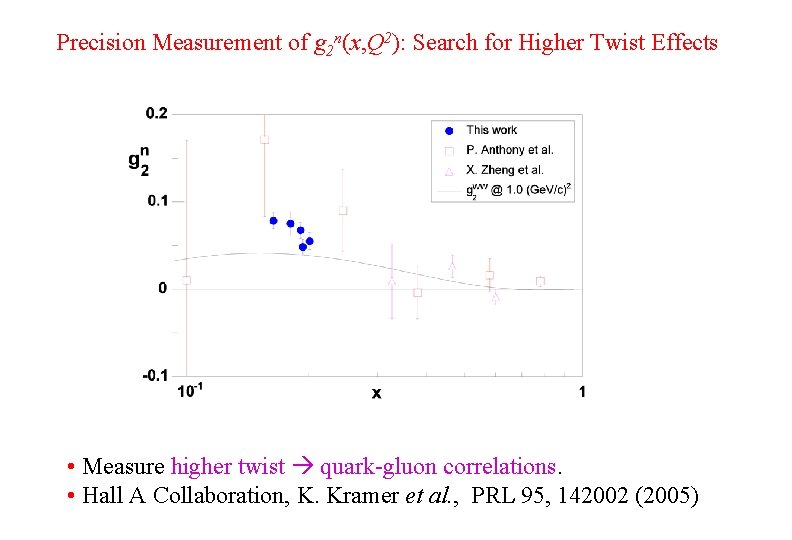
Precision Measurement of g 2 n(x, Q 2): Search for Higher Twist Effects • Measure higher twist quark-gluon correlations. • Hall A Collaboration, K. Kramer et al. , PRL 95, 142002 (2005)
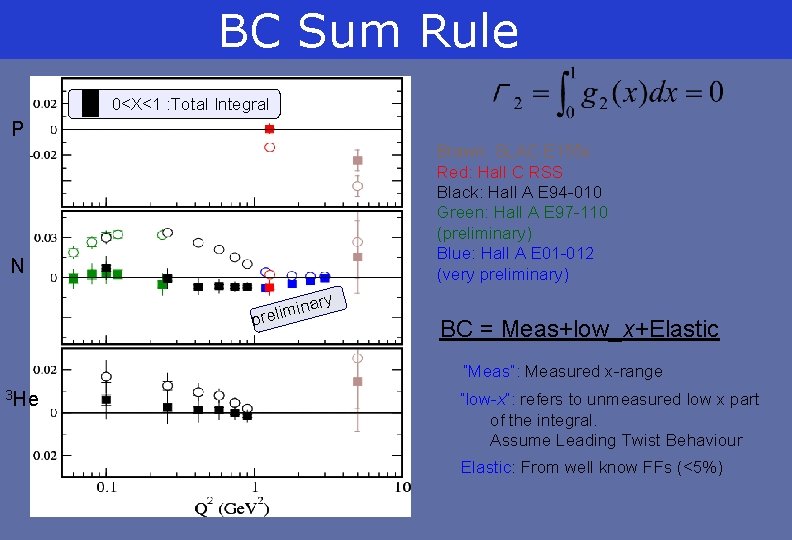
BC Sum Rule 0<X<1 : Total Integral P Brawn: SLAC E 155 x Red: Hall C RSS Black: Hall A E 94 -010 Green: Hall A E 97 -110 (preliminary) Blue: Hall A E 01 -012 (very preliminary) N ary in prelim BC = Meas+low_x+Elastic “Meas”: Measured x-range 3 He “low-x”: refers to unmeasured low x part of the integral. Assume Leading Twist Behaviour Elastic: From well know FFs (<5%)
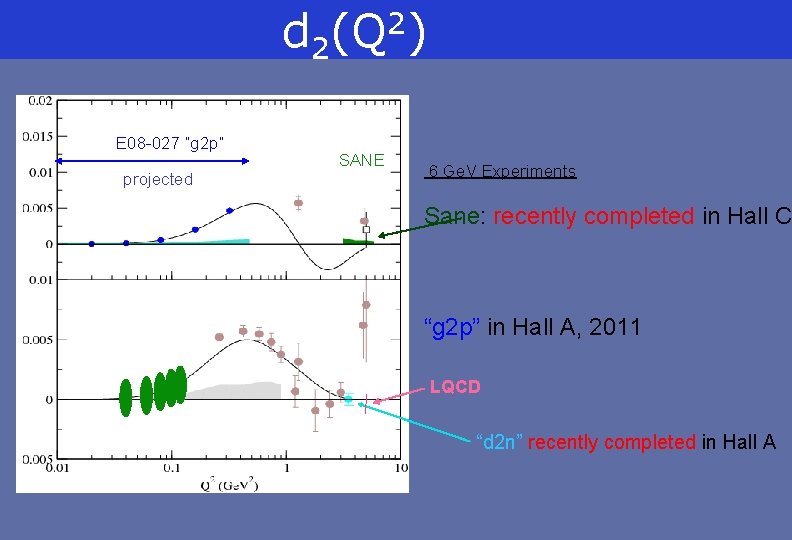
d 2(Q 2) E 08 -027 “g 2 p” projected SANE 6 Ge. V Experiments Sane: recently completed in Hall C “g 2 p” in Hall A, 2011 LQCD “d 2 n” recently completed in Hall A
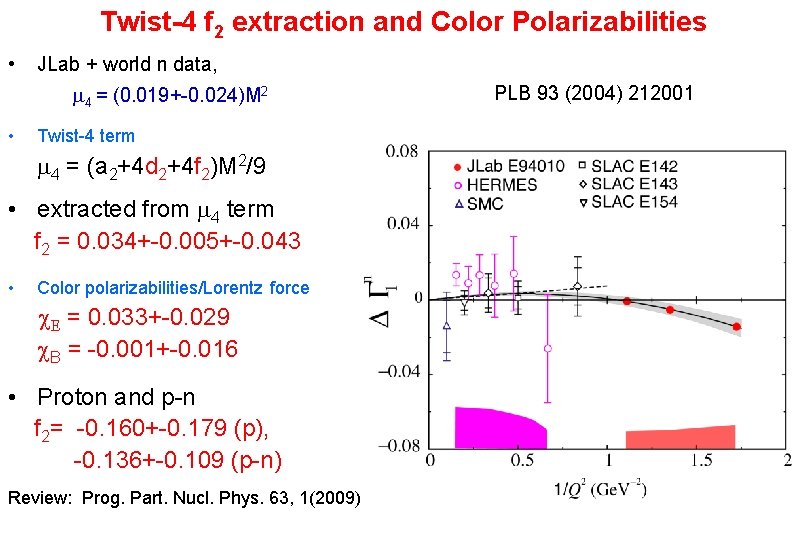
Twist-4 f 2 extraction and Color Polarizabilities • JLab + world n data, m 4 = (0. 019+-0. 024)M 2 • Twist-4 term m 4 = (a 2+4 d 2+4 f 2)M 2/9 • extracted from m 4 term f 2 = 0. 034+-0. 005+-0. 043 • Color polarizabilities/Lorentz force c. E = 0. 033+-0. 029 c. B = -0. 001+-0. 016 • Proton and p-n f 2= -0. 160+-0. 179 (p), -0. 136+-0. 109 (p-n) Review: Prog. Part. Nucl. Phys. 63, 1(2009) PLB 93 (2004) 212001
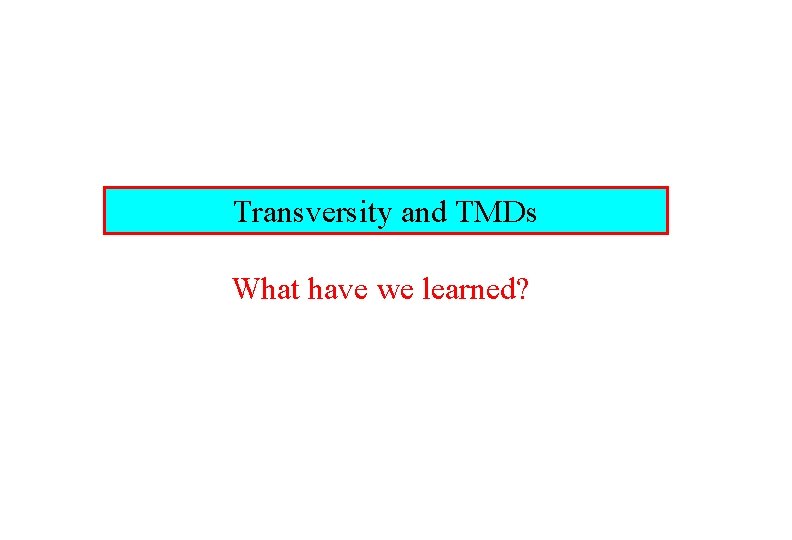
Transversity and TMDs What have we learned?
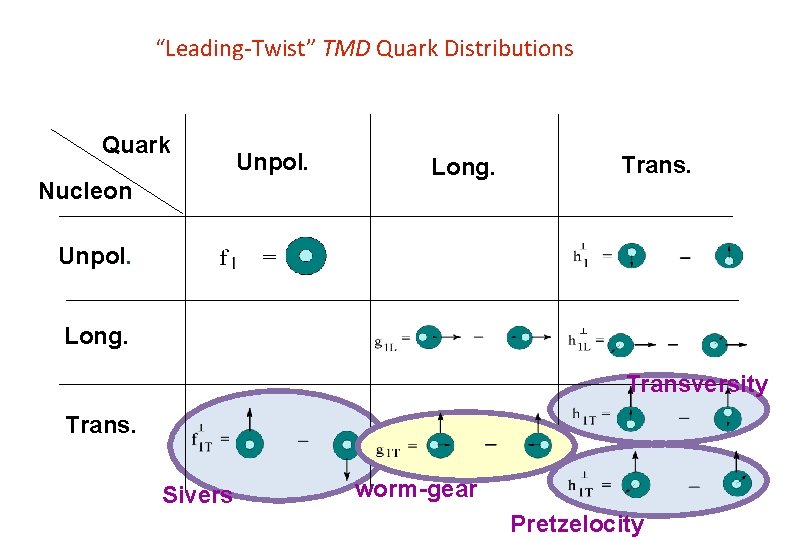
“Leading-Twist” TMD Quark Distributions Quark Nucleon Unpol. Long. Trans. Unpol. Long. Transversity Trans. Sivers worm-gear Pretzelocity
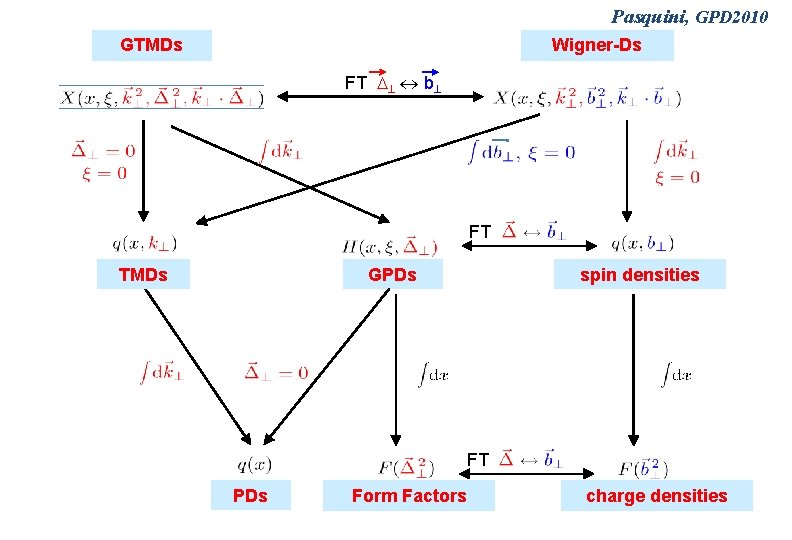
Pasquini, GPD 2010 GTMDs Wigner-Ds FT b FT TMDs GPDs spin densities FT PDs Form Factors charge densities
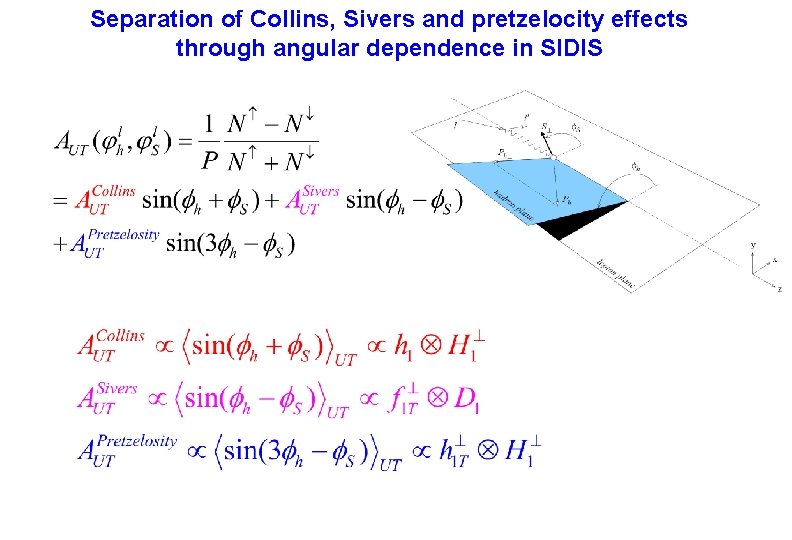
Separation of Collins, Sivers and pretzelocity effects through angular dependence in SIDIS
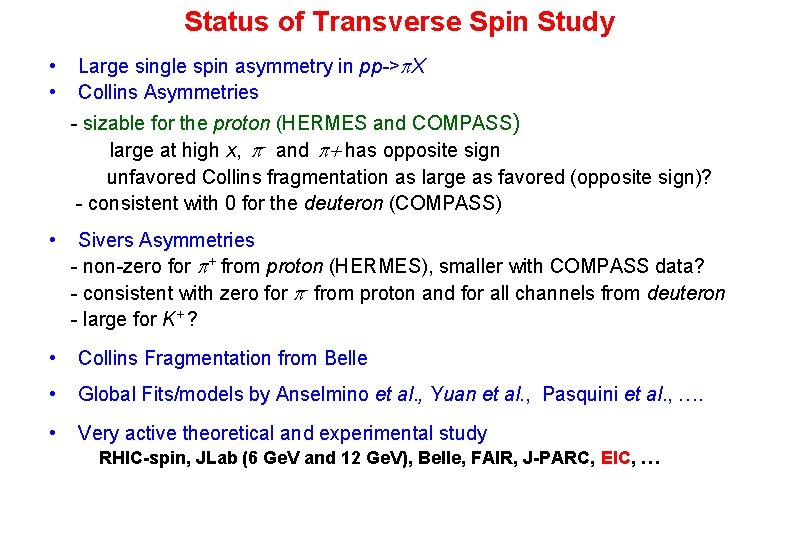
Status of Transverse Spin Study • • Large single spin asymmetry in pp->p. X Collins Asymmetries - sizable for the proton (HERMES and COMPASS) large at high x, p- and p+ has opposite sign unfavored Collins fragmentation as large as favored (opposite sign)? - consistent with 0 for the deuteron (COMPASS) • Sivers Asymmetries - non-zero for p+ from proton (HERMES), smaller with COMPASS data? - consistent with zero for p- from proton and for all channels from deuteron - large for K+ ? • Collins Fragmentation from Belle • Global Fits/models by Anselmino et al. , Yuan et al. , Pasquini et al. , …. • Very active theoretical and experimental study RHIC-spin, JLab (6 Ge. V and 12 Ge. V), Belle, FAIR, J-PARC, EIC, …
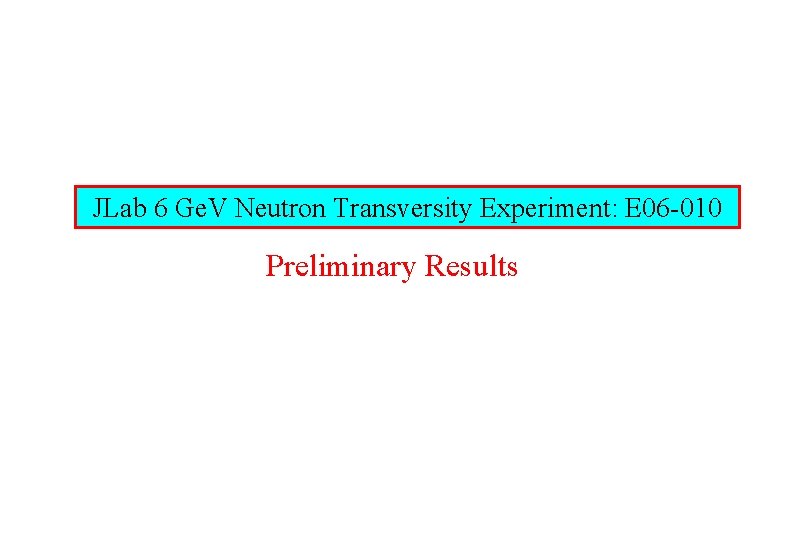
JLab 6 Ge. V Neutron Transversity Experiment: E 06 -010 Preliminary Results
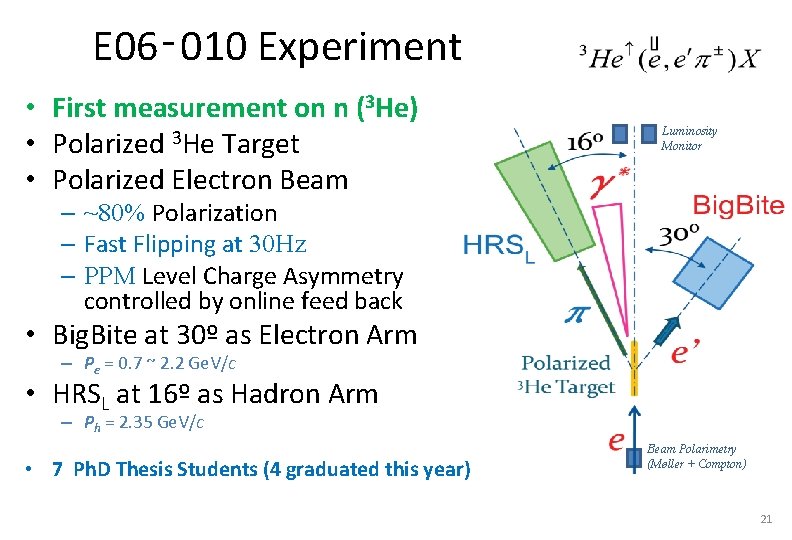
E 06‑ 010 Experiment • First measurement on n (3 He) • Polarized 3 He Target • Polarized Electron Beam Luminosity Monitor – ~80% Polarization – Fast Flipping at 30 Hz – PPM Level Charge Asymmetry controlled by online feed back • Big. Bite at 30º as Electron Arm – Pe = 0. 7 ~ 2. 2 Ge. V/c • HRSL at 16º as Hadron Arm – Ph = 2. 35 Ge. V/c • 7 Ph. D Thesis Students (4 graduated this year) Beam Polarimetry (Møller + Compton) 21
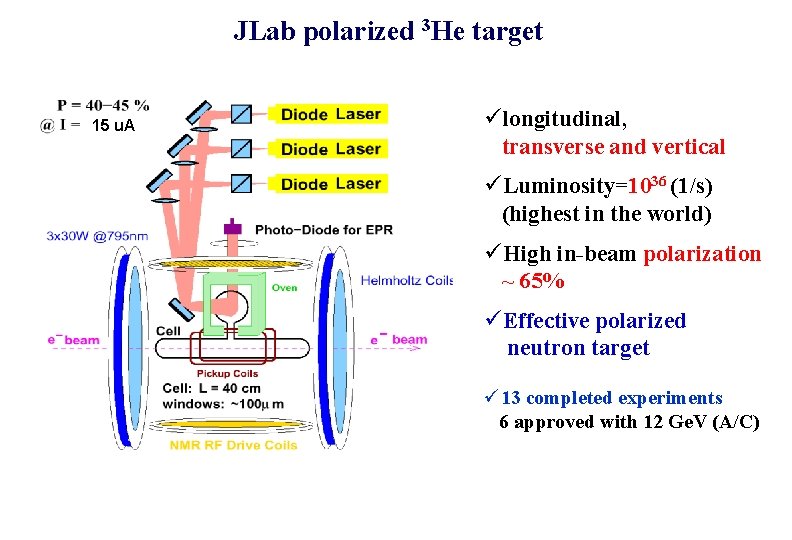
JLab polarized 3 He target 15 u. A ülongitudinal, transverse and vertical üLuminosity=1036 (1/s) (highest in the world) üHigh in-beam polarization ~ 65% üEffective polarized neutron target ü 13 completed experiments 6 approved with 12 Ge. V (A/C)
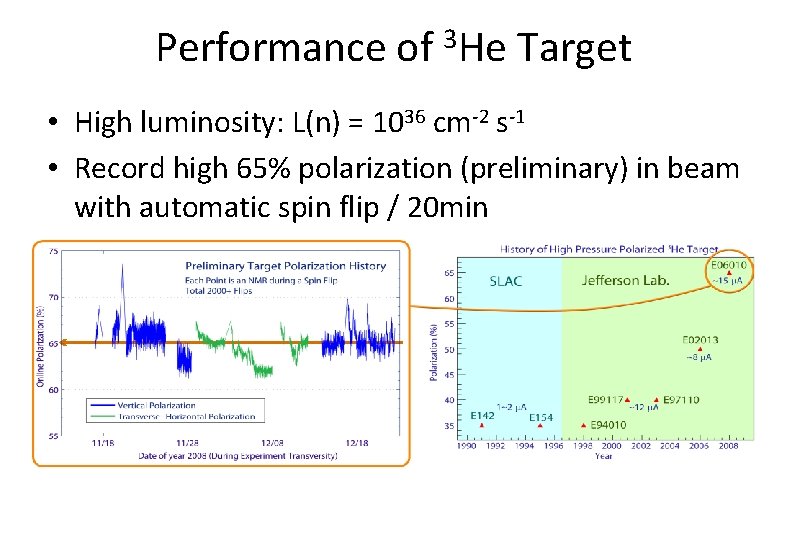
Performance of 3 He Target • High luminosity: L(n) = 1036 cm-2 s-1 • Record high 65% polarization (preliminary) in beam with automatic spin flip / 20 min
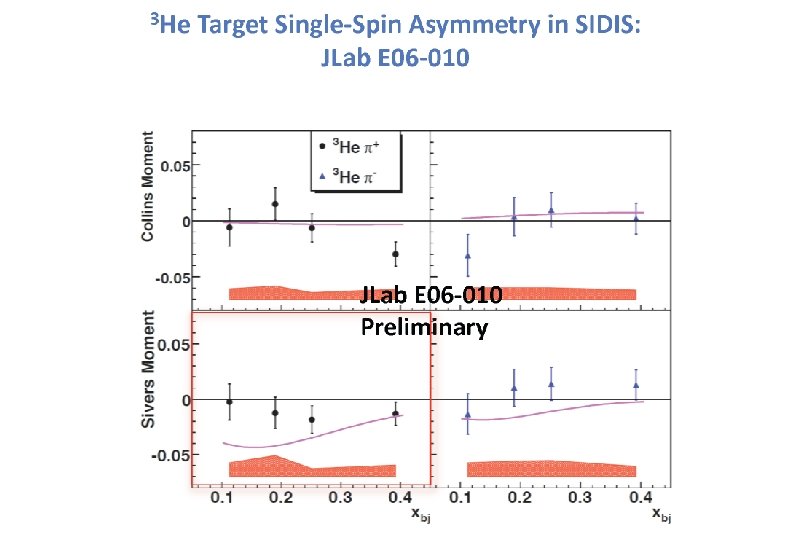
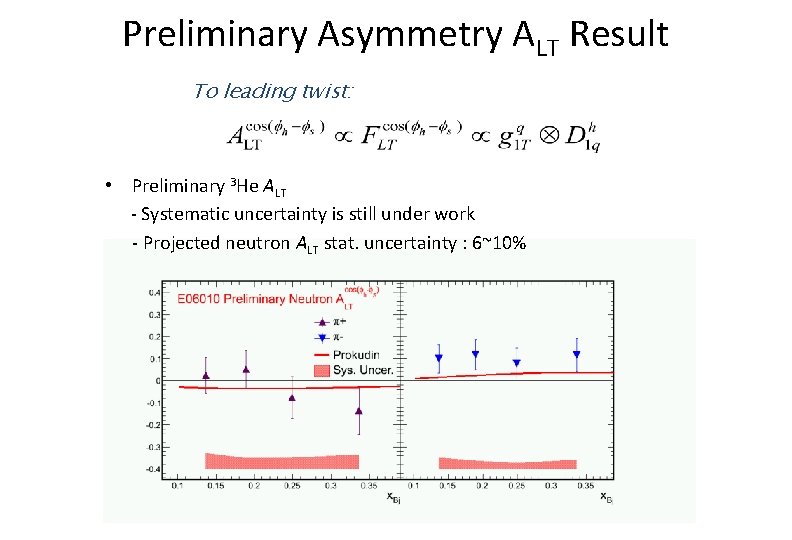
Preliminary Asymmetry ALT Result To leading twist: • Preliminary 3 He ALT - Systematic uncertainty is still under work - Projected neutron ALT stat. uncertainty : 6~10%
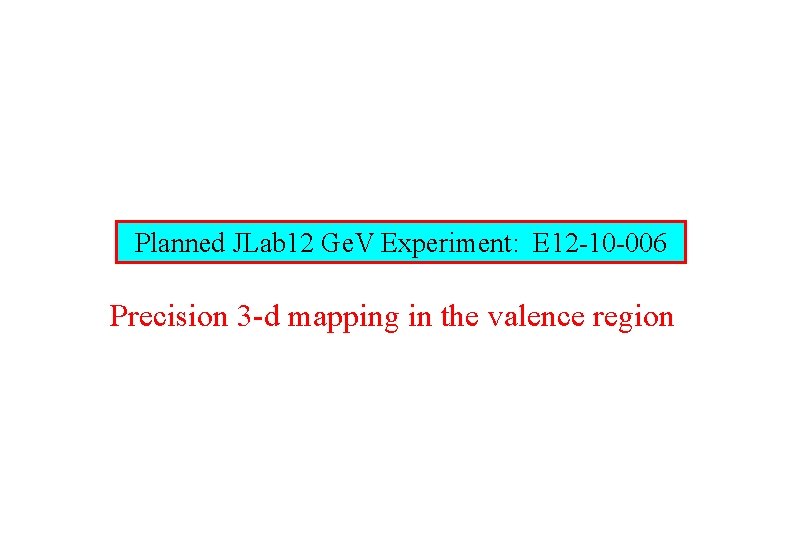
Planned JLab 12 Ge. V Experiment: E 12 -10 -006 Precision 3 -d mapping in the valence region
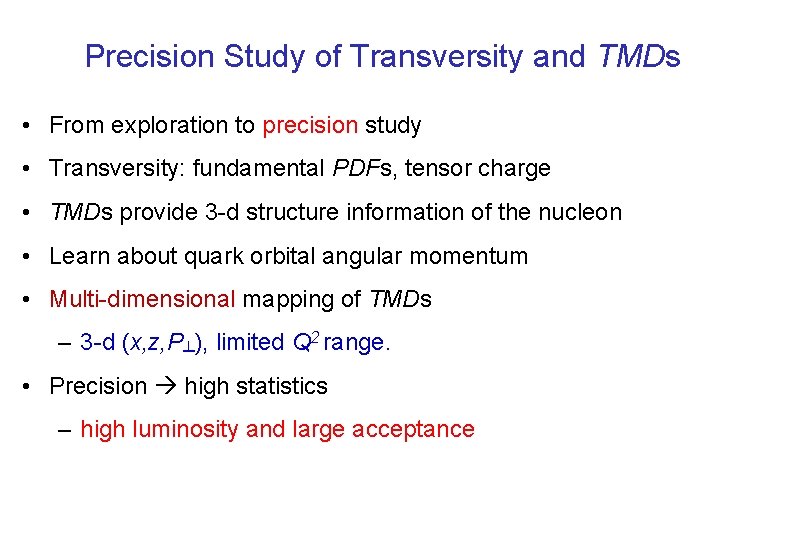
Precision Study of Transversity and TMDs • From exploration to precision study • Transversity: fundamental PDFs, tensor charge • TMDs provide 3 -d structure information of the nucleon • Learn about quark orbital angular momentum • Multi-dimensional mapping of TMDs – 3 -d (x, z, P┴), limited Q 2 range. • Precision high statistics – high luminosity and large acceptance
![Solenoid detector for SIDIS at 11 Ge V Ycm Yoke Coil 3 He Target Solenoid detector for SIDIS at 11 Ge. V Y[cm] Yoke Coil 3 He Target](https://slidetodoc.com/presentation_image_h2/f2e985179333e1a0b1e4396482423626/image-28.jpg)
Solenoid detector for SIDIS at 11 Ge. V Y[cm] Yoke Coil 3 He Target FGEMx 4 Aerogel LGEMx 4 LS Gas Chere nkov HG SH GEMx 2 PS Z[cm]
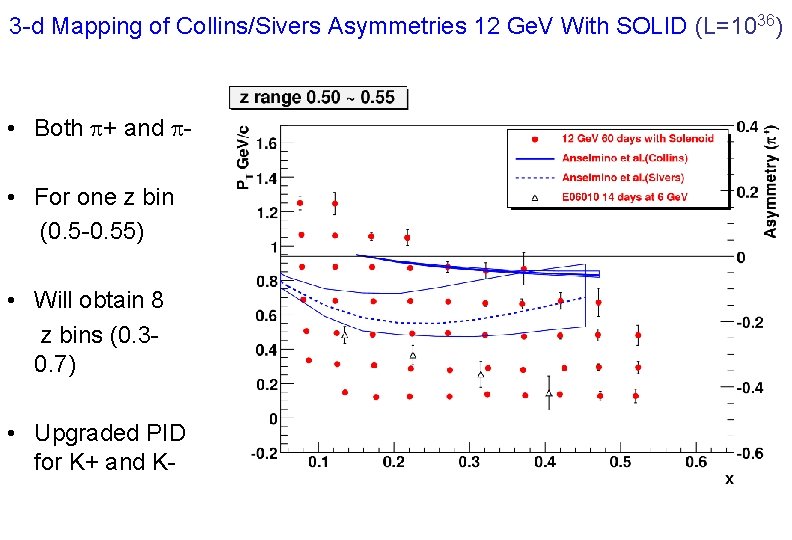
3 -d Mapping of Collins/Sivers Asymmetries 12 Ge. V With SOLID (L=1036) • Both p+ and p • For one z bin (0. 5 -0. 55) • Will obtain 8 z bins (0. 30. 7) • Upgraded PID for K+ and K-
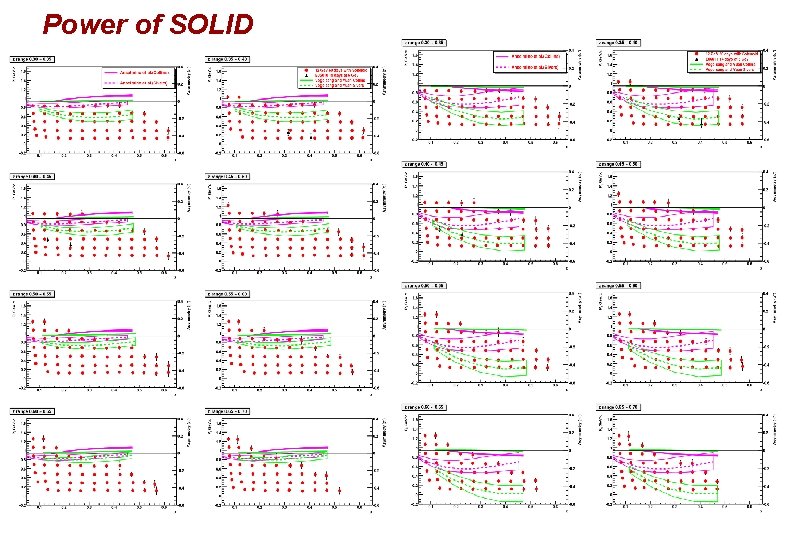
Power of SOLID
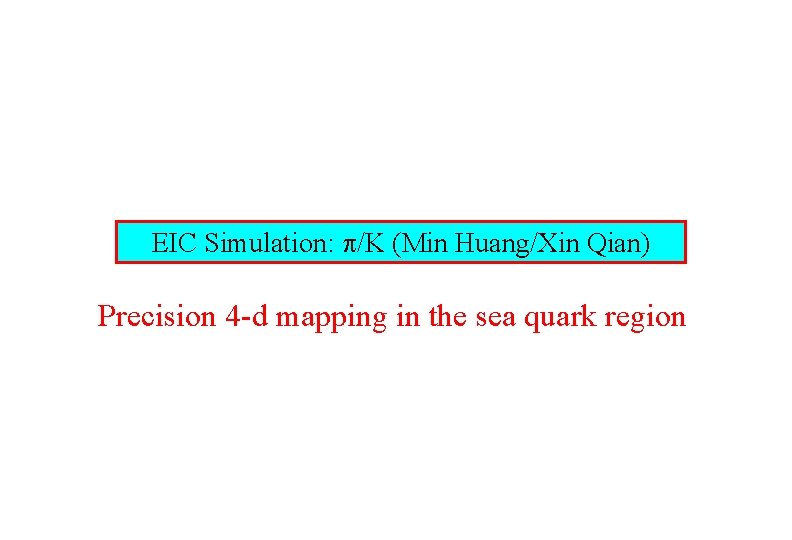
EIC Simulation: p/K (Min Huang/Xin Qian) Precision 4 -d mapping in the sea quark region
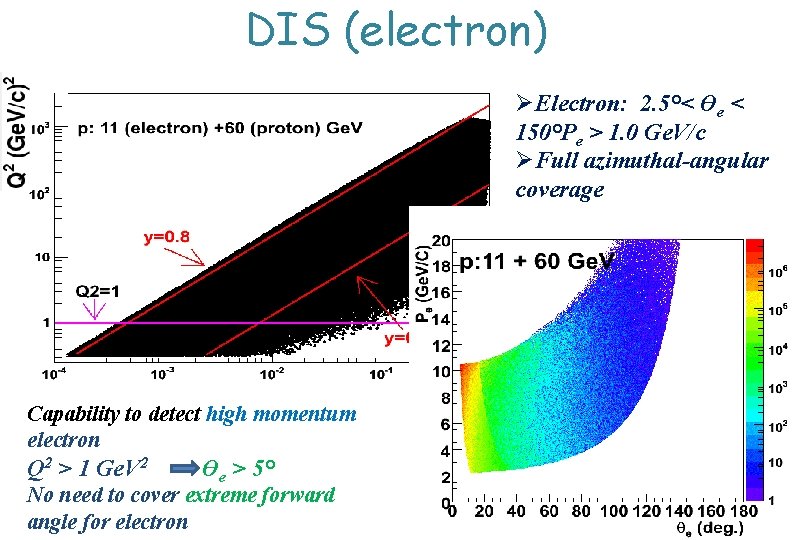
DIS (electron) ØElectron: 2. 5°< ϴe < 150°Pe > 1. 0 Ge. V/c ØFull azimuthal-angular coverage DIS cut: Q 2 > 1 Ge. V 2 W > 2. 3 Ge. V 0. 8 > y > 0. 05 Capability to detect high momentum electron Q 2 > 1 Ge. V 2 ϴe > 5° No need to cover extreme forward angle for electron
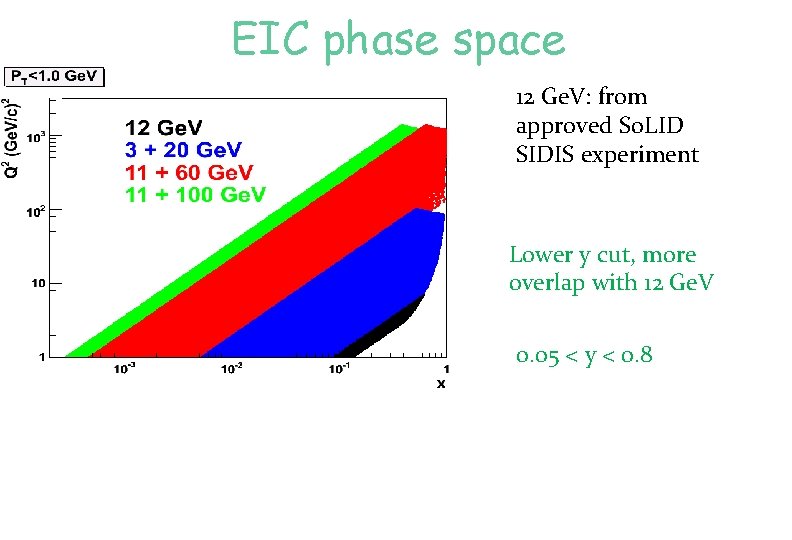
EIC phase space 12 Ge. V: from approved So. LID SIDIS experiment Lower y cut, more overlap with 12 Ge. V 0. 05 < y < 0. 8
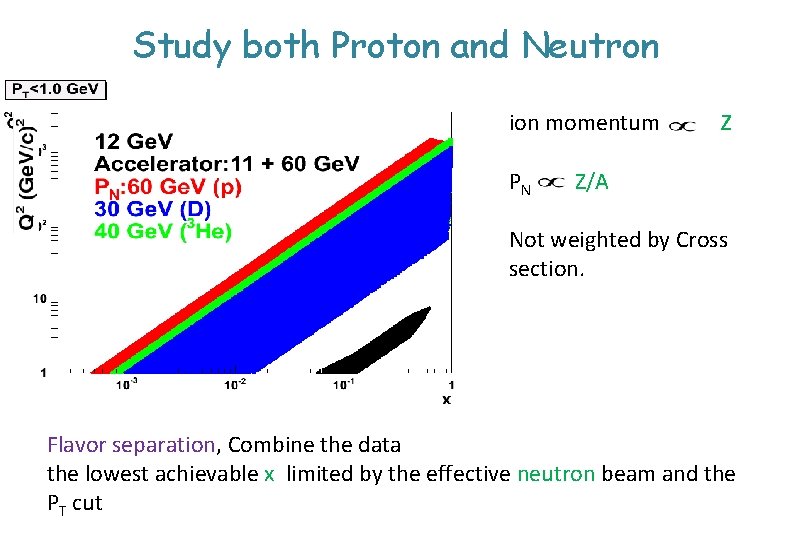
Study both Proton and Neutron ion momentum PN z Z/A Not weighted by Cross section. Flavor separation, Combine the data the lowest achievable x limited by the effective neutron beam and the PT cut
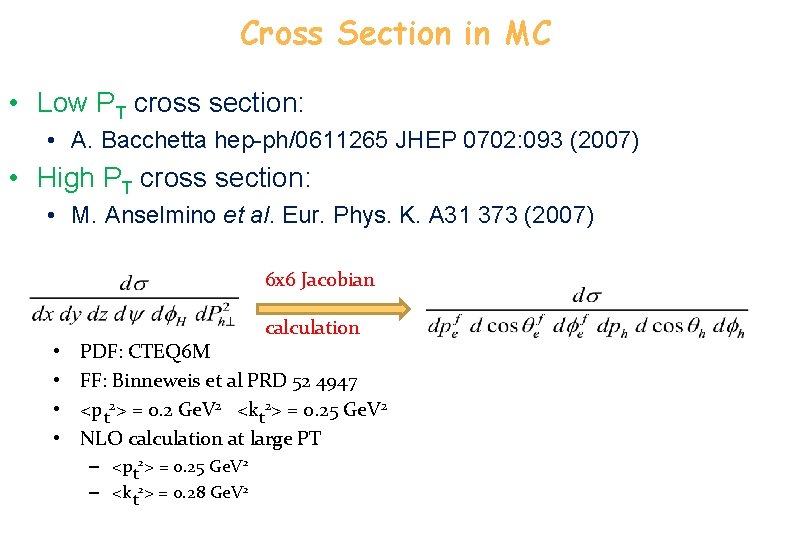
Cross Section in MC • Low PT cross section: • A. Bacchetta hep-ph/0611265 JHEP 0702: 093 (2007) • High PT cross section: • M. Anselmino et al. Eur. Phys. K. A 31 373 (2007) 6 x 6 Jacobian calculation • • PDF: CTEQ 6 M FF: Binneweis et al PRD 52 4947 <pt 2> = 0. 2 Ge. V 2 <kt 2> = 0. 25 Ge. V 2 NLO calculation at large PT – <pt 2> = 0. 25 Ge. V 2 – <kt 2> = 0. 28 Ge. V 2
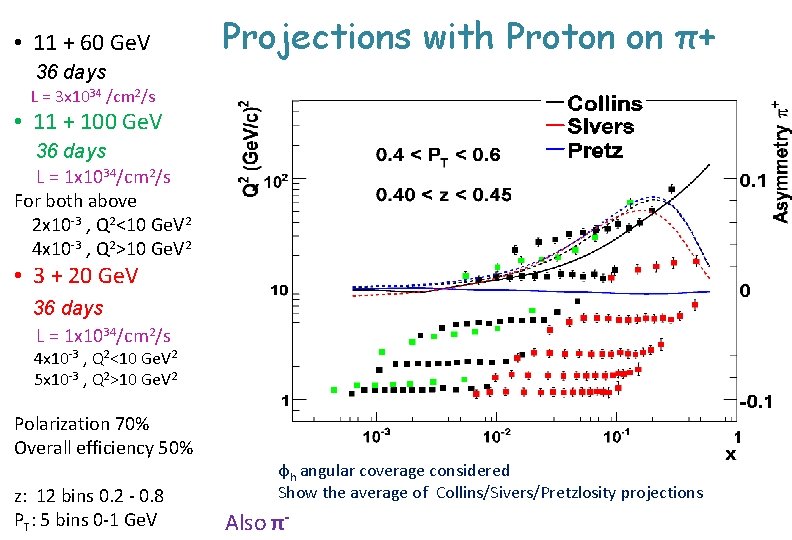
• 11 + 60 Ge. V Projections with Proton on π+ 36 days L = 3 x 1034 /cm 2/s • 11 + 100 Ge. V 36 days L = 1 x 1034/cm 2/s For both above 2 x 10 -3 , Q 2<10 Ge. V 2 4 x 10 -3 , Q 2>10 Ge. V 2 • 3 + 20 Ge. V 36 days L = 1 x 1034/cm 2/s 4 x 10 -3 , Q 2<10 Ge. V 2 5 x 10 -3 , Q 2>10 Ge. V 2 Polarization 70% Overall efficiency 50% z: 12 bins 0. 2 - 0. 8 PT: 5 bins 0 -1 Ge. V φh angular coverage considered Show the average of Collins/Sivers/Pretzlosity projections Also π-
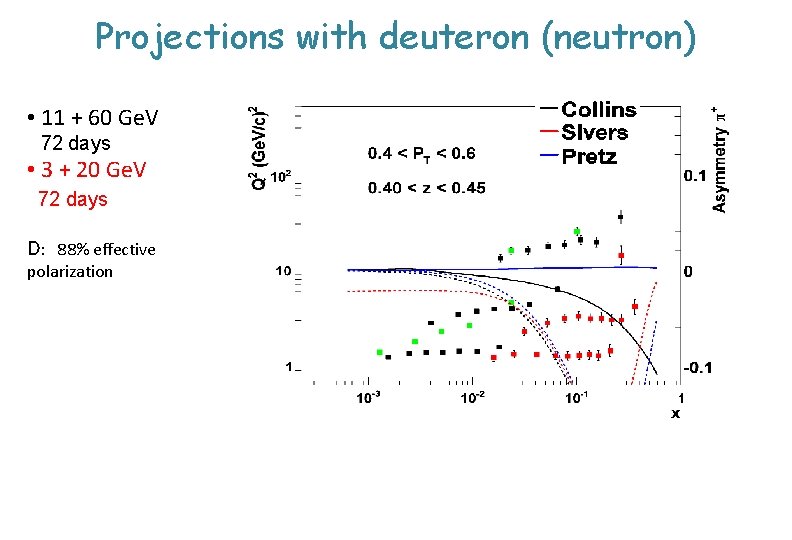
Projections with deuteron (neutron) • 11 + 60 Ge. V 72 days • 3 + 20 Ge. V 72 days D: 88% effective polarization
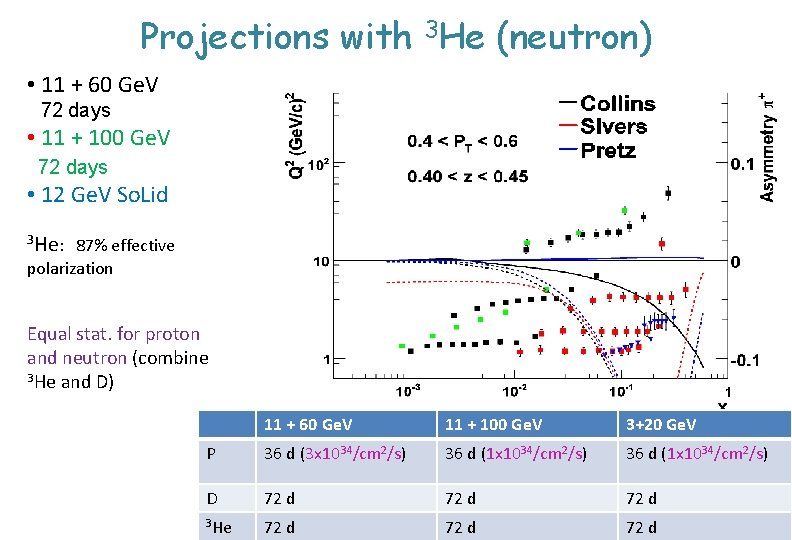
Projections with 3 He (neutron) • 11 + 60 Ge. V 72 days • 11 + 100 Ge. V 72 days • 12 Ge. V So. Lid 3 He: 87% effective polarization Equal stat. for proton and neutron (combine 3 He and D) 11 + 60 Ge. V 11 + 100 Ge. V 3+20 Ge. V P 36 d (3 x 1034/cm 2/s) 36 d (1 x 1034/cm 2/s) D 72 d 3 He 72 d
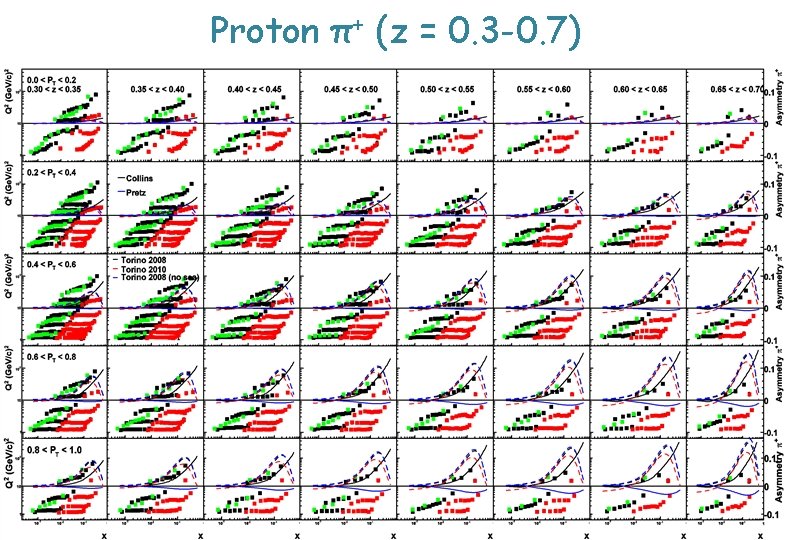
Proton π+ (z = 0. 3 -0. 7)
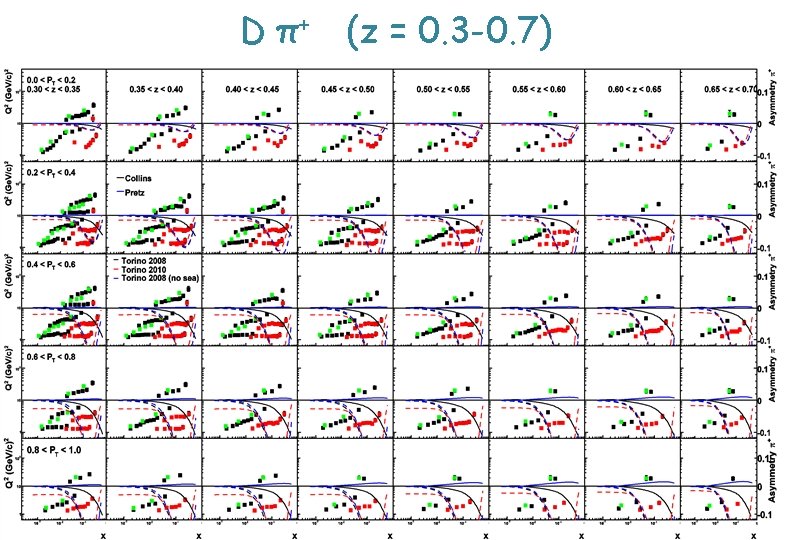
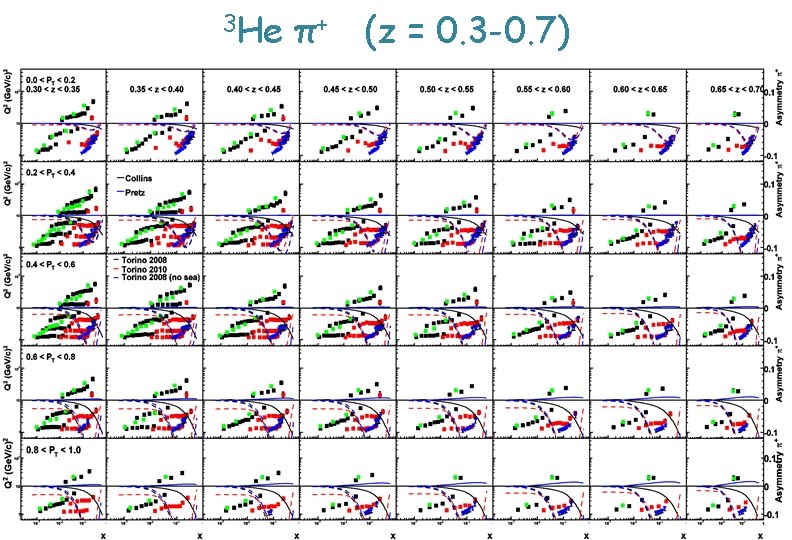
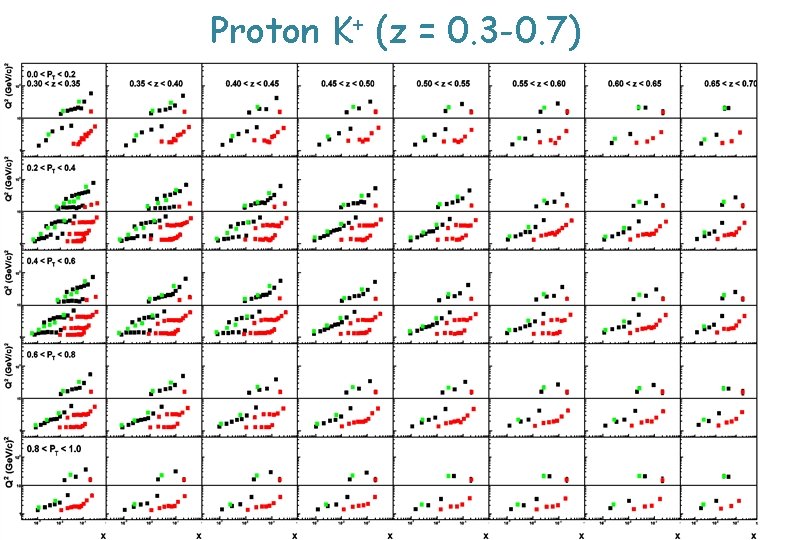
Proton K+ (z = 0. 3 -0. 7)
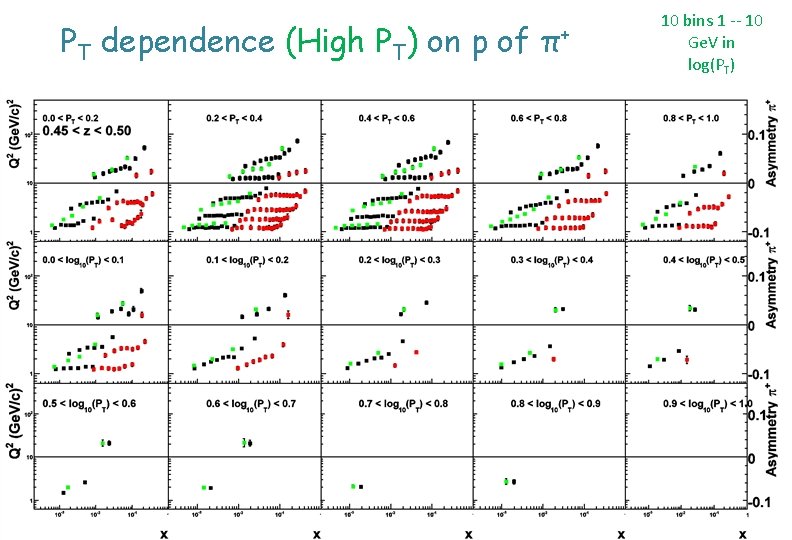
PT dependence (High PT) on p of π+ 10 bins 1 -- 10 Ge. V in log(PT)
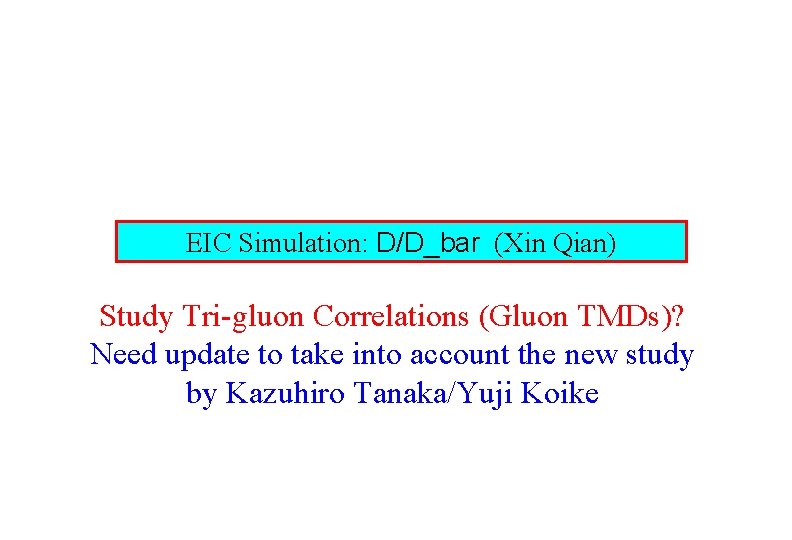
EIC Simulation: D/D_bar (Xin Qian) Study Tri-gluon Correlations (Gluon TMDs)? Need update to take into account the new study by Kazuhiro Tanaka/Yuji Koike
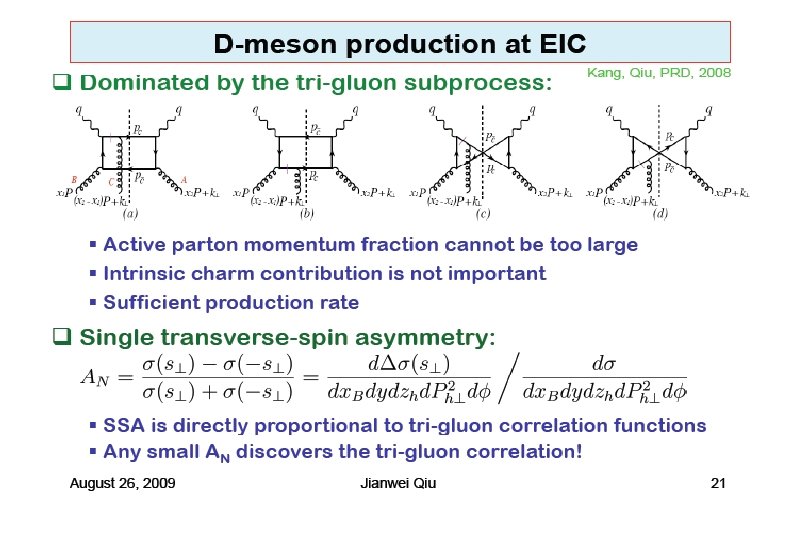
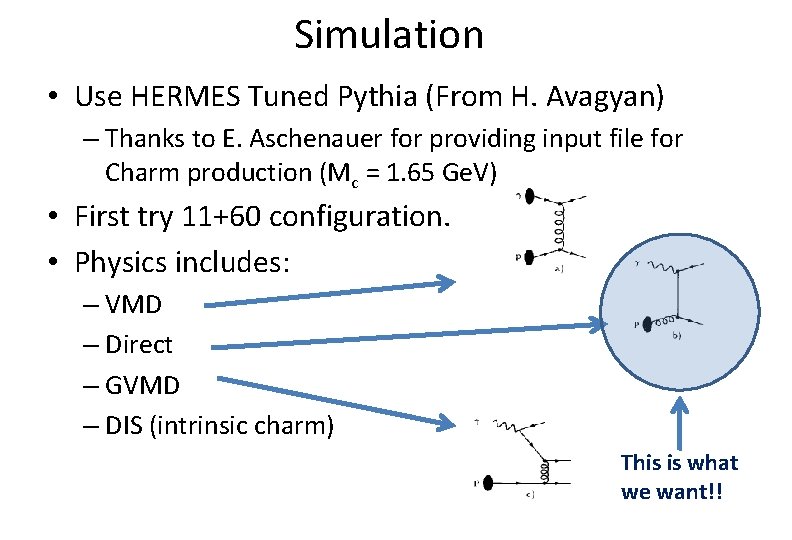
Simulation • Use HERMES Tuned Pythia (From H. Avagyan) – Thanks to E. Aschenauer for providing input file for Charm production (Mc = 1. 65 Ge. V) • First try 11+60 configuration. • Physics includes: – VMD – Direct – GVMD – DIS (intrinsic charm) This is what we want!!
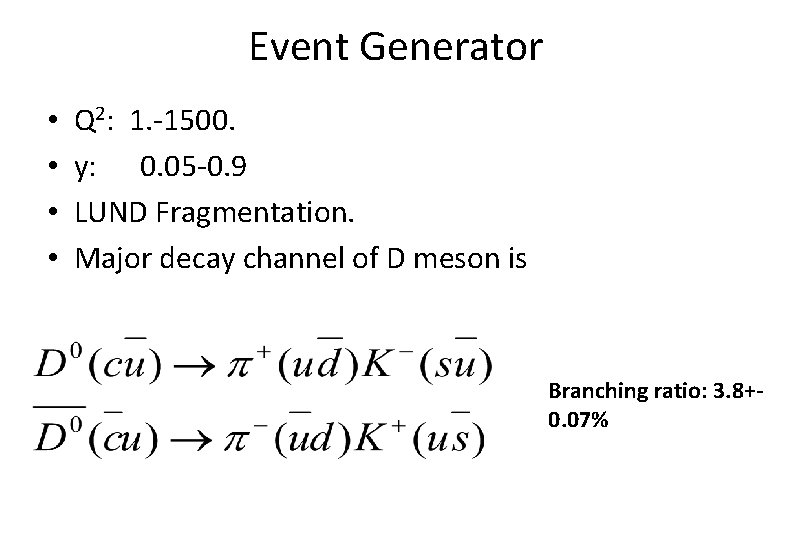
Event Generator • • Q 2: 1. -1500. y: 0. 05 -0. 9 LUND Fragmentation. Major decay channel of D meson is Branching ratio: 3. 8+0. 07%
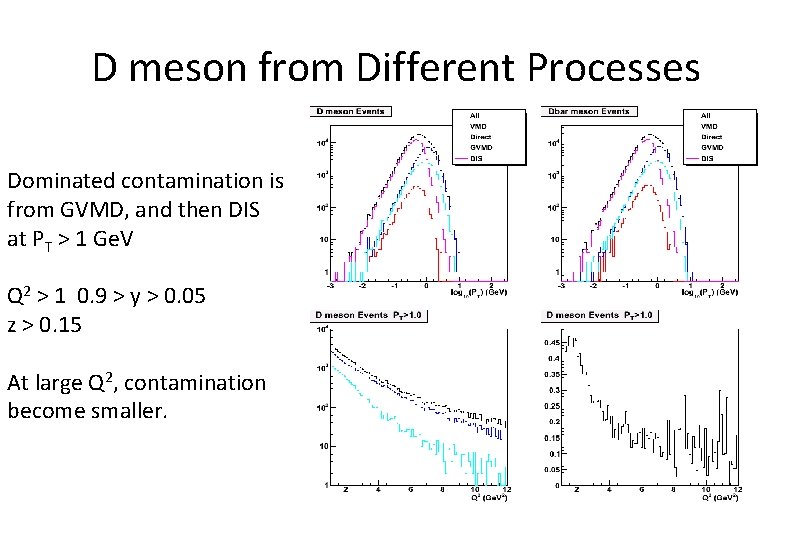
D meson from Different Processes Dominated contamination is from GVMD, and then DIS at PT > 1 Ge. V Q 2 > 1 0. 9 > y > 0. 05 z > 0. 15 At large Q 2, contamination become smaller.
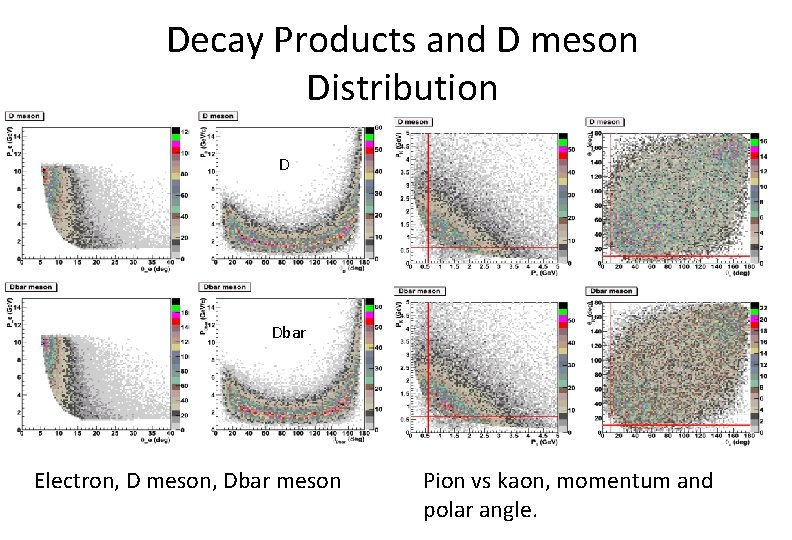
Decay Products and D meson Distribution D Dbar Electron, D meson, Dbar meson Pion vs kaon, momentum and polar angle.
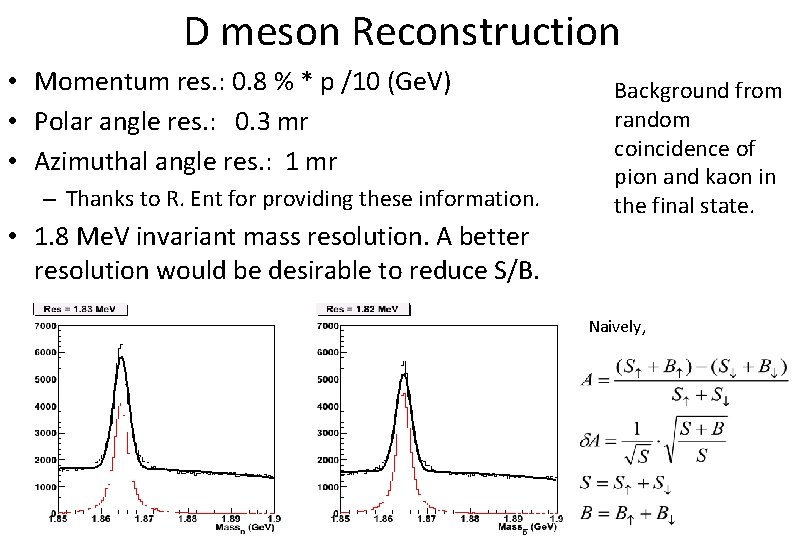
D meson Reconstruction • Momentum res. : 0. 8 % * p /10 (Ge. V) • Polar angle res. : 0. 3 mr • Azimuthal angle res. : 1 mr – Thanks to R. Ent for providing these information. • 1. 8 Me. V invariant mass resolution. A better resolution would be desirable to reduce S/B. Background from random coincidence of pion and kaon in the final state. Naively,
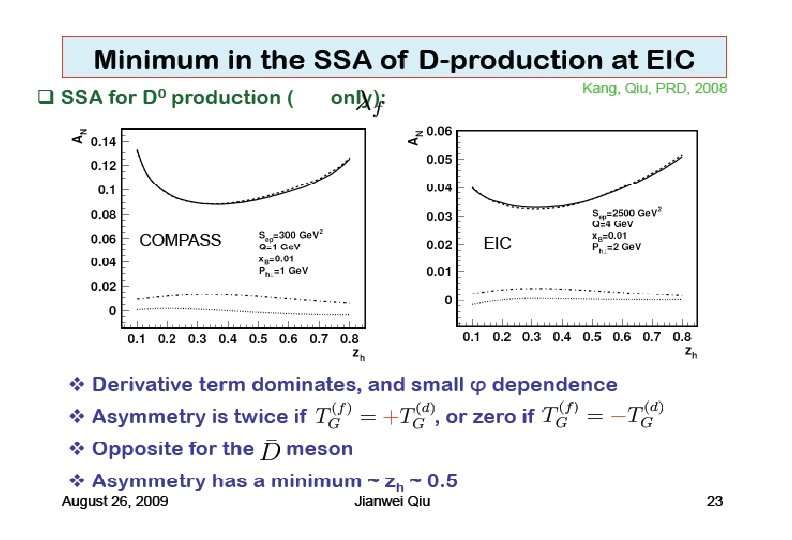
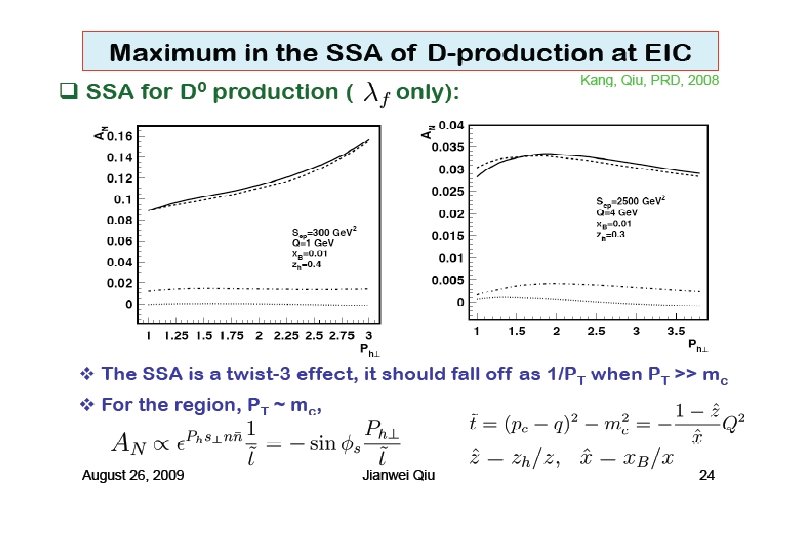
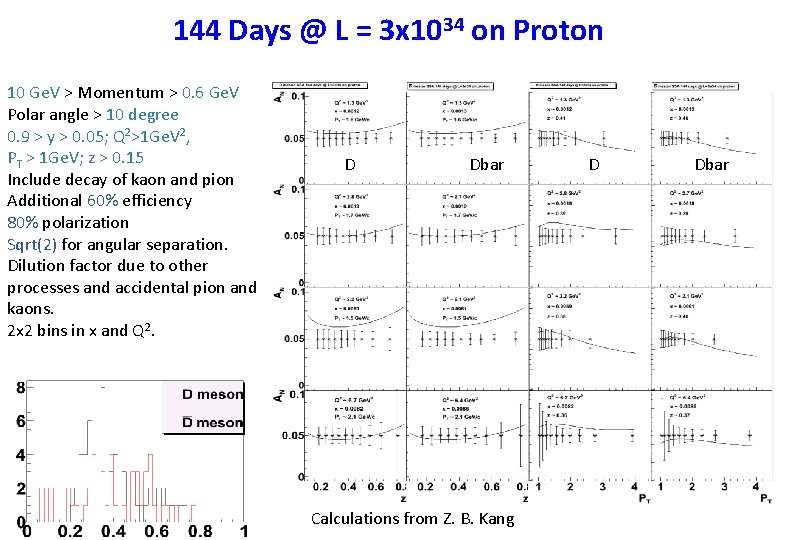
144 Days @ L = 3 x 1034 on Proton 10 Ge. V > Momentum > 0. 6 Ge. V Polar angle > 10 degree 0. 9 > y > 0. 05; Q 2>1 Ge. V 2, PT > 1 Ge. V; z > 0. 15 Include decay of kaon and pion Additional 60% efficiency 80% polarization Sqrt(2) for angular separation. Dilution factor due to other processes and accidental pion and kaons. 2 x 2 bins in x and Q 2. D Dbar Calculations from Z. B. Kang D Dbar
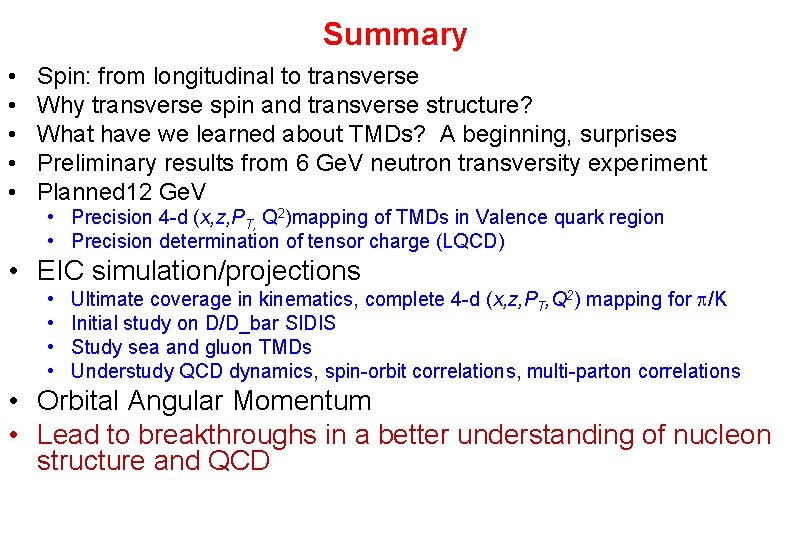
Summary • • • Spin: from longitudinal to transverse Why transverse spin and transverse structure? What have we learned about TMDs? A beginning, surprises Preliminary results from 6 Ge. V neutron transversity experiment Planned 12 Ge. V • Precision 4 -d (x, z, PT, Q 2)mapping of TMDs in Valence quark region • Precision determination of tensor charge (LQCD) • EIC simulation/projections • • Ultimate coverage in kinematics, complete 4 -d (x, z, PT, Q 2) mapping for p/K Initial study on D/D_bar SIDIS Study sea and gluon TMDs Understudy QCD dynamics, spin-orbit correlations, multi-parton correlations • Orbital Angular Momentum • Lead to breakthroughs in a better understanding of nucleon structure and QCD
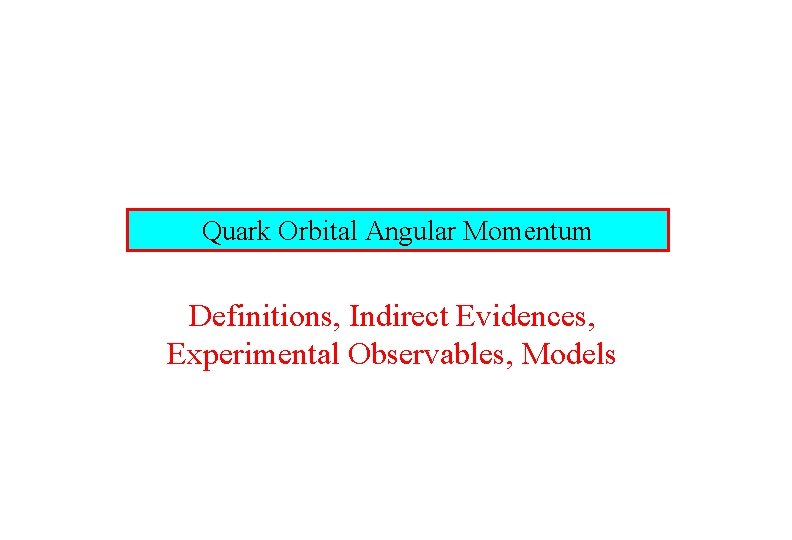
Quark Orbital Angular Momentum Definitions, Indirect Evidences, Experimental Observables, Models
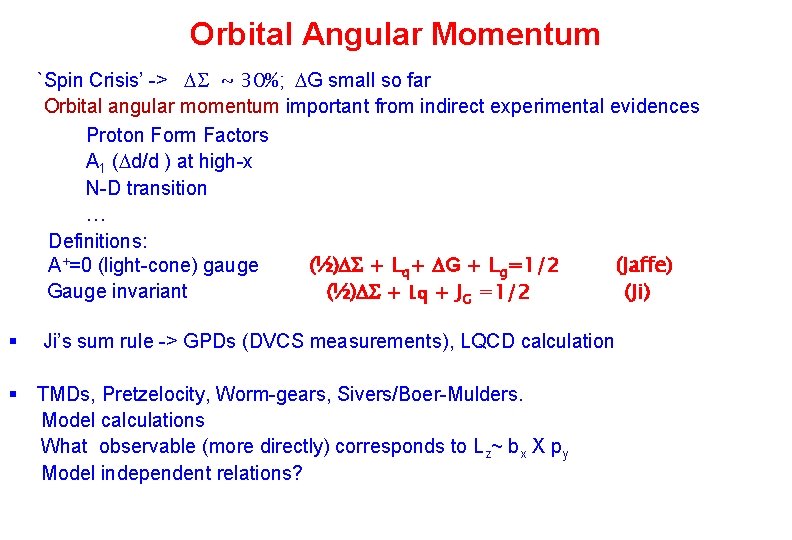
Orbital Angular Momentum `Spin Crisis’ -> ~ 30%; G small so far Orbital angular momentum important from indirect experimental evidences Proton Form Factors A 1 ( d/d ) at high-x N-D transition … Definitions: A+=0 (light-cone) gauge (½)DS + Lq+ DG + Lg=1/2 (Jaffe) Gauge invariant (½)DS + Lq + JG =1/2 (Ji) § Ji’s sum rule -> GPDs (DVCS measurements), LQCD calculation § TMDs, Pretzelocity, Worm-gears, Sivers/Boer-Mulders. Model calculations What observable (more directly) corresponds to Lz~ bx X py Model independent relations?
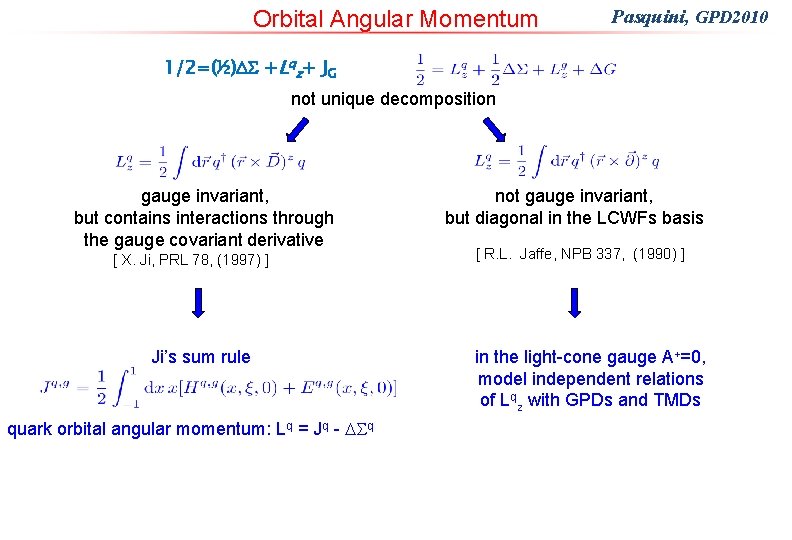
Orbital Angular Momentum Pasquini, GPD 2010 1/2=(½)DS +Lqz+ JG not unique decomposition gauge invariant, but contains interactions through the gauge covariant derivative [ X. Ji, PRL 78, (1997) ] Ji’s sum rule quark orbital angular momentum: Lq = Jq - q not gauge invariant, but diagonal in the LCWFs basis [ R. L. Jaffe, NPB 337, (1990) ] in the light-cone gauge A+=0, model independent relations of Lqz with GPDs and TMDs
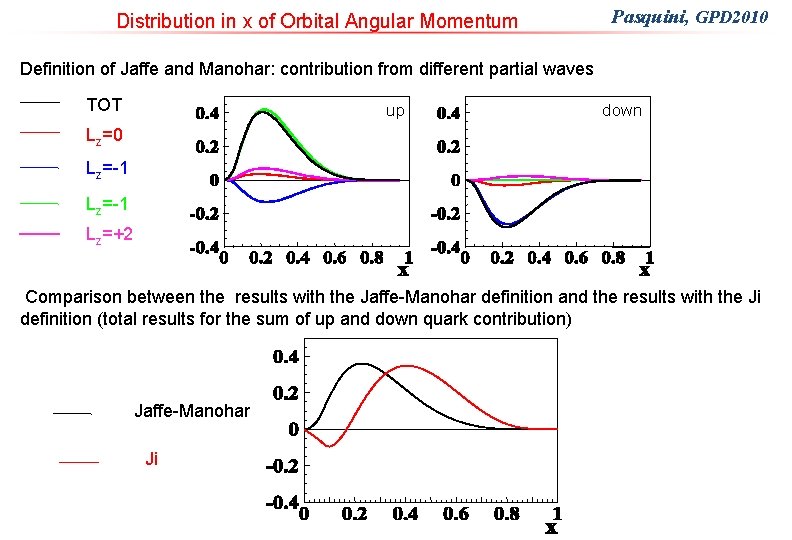
Distribution in x of Orbital Angular Momentum Pasquini, GPD 2010 Definition of Jaffe and Manohar: contribution from different partial waves TOT up down Lz=0 Lz=-1 Lz=+2 Comparison between the results with the Jaffe-Manohar definition and the results with the Ji definition (total results for the sum of up and down quark contribution) Jaffe-Manohar Ji
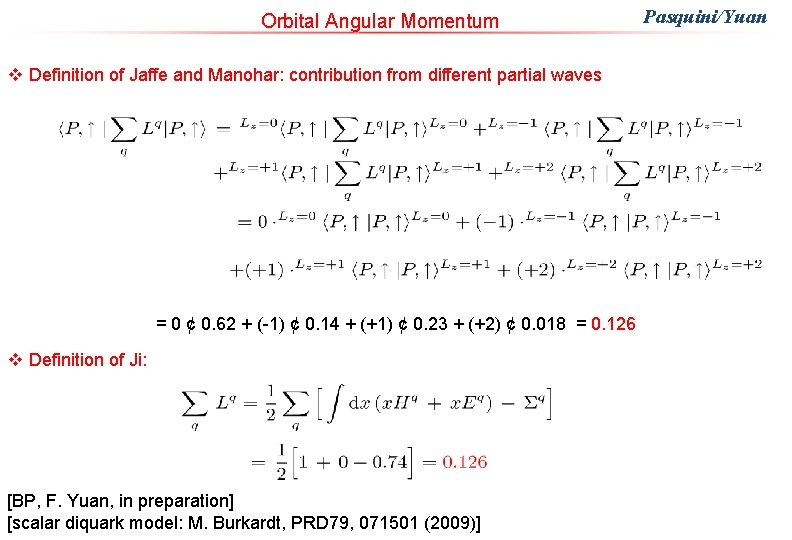
Orbital Angular Momentum v Definition of Jaffe and Manohar: contribution from different partial waves = 0 ¢ 0. 62 + (-1) ¢ 0. 14 + (+1) ¢ 0. 23 + (+2) ¢ 0. 018 = 0. 126 v Definition of Ji: [BP, F. Yuan, in preparation] [scalar diquark model: M. Burkardt, PRD 79, 071501 (2009)] Pasquini/Yuan
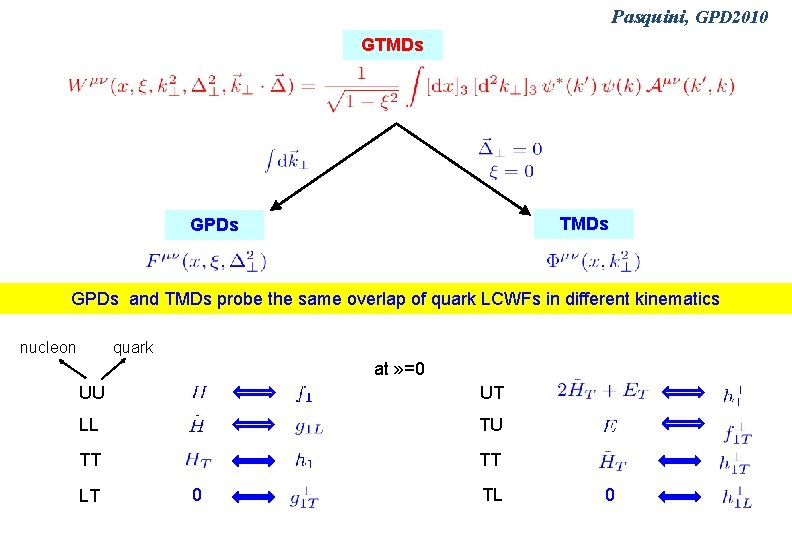
Pasquini, GPD 2010 GTMDs GPDs and TMDs probe the same overlap of quark LCWFs in different kinematics nucleon quark at » =0 UU UT LL TU TT TT LT 0 TL 0
Unpold
Tmds
Low spin and high spin complex
Geminal coupling
Spin spin coupling
Spin spin coupling
優點
Ligaments of the stomach
Advantage and disadvantage of transverse framing
Longitudinal and transverse framing system
Draw and label transverse wave
Transverse and longitudnal waves
Spin glasses and complexity
Optimal driver launch angle and spin rate chart
What is the last
Divine intervention podcast biostatistics
Length of transverse axis of hyperbola
How to measure ap diameter of chest
Conjugate and transverse axis
Smooth muscle
Wave unit test answer key
Pericardium layers
Wavelength formula triangle
Can you recover from a flat spin
Tempo e tecnologia
Implicatievragen voorbeeld
New spin
Define spin selling
Spin finish oil formulation
Specificity spin
High surface tension vs low surface tension
Spin selection rule
Modificação
Qumica
Magnetic quantum number of nitrogen
Spin quantum number
What is spin plan
Spin 1 operators
Spin coating speed
Selection rule for electronic transition
Spin-fin kysely
Spin of hydrogen nucleus is
Nmr principle
Spin positivo y negativo
Spin off significato
Conclusion of operating system
Selection rule for electronic transition
Floch
Spin selection rule
Spin echo
Hundsche regel
Disc filters galaxy 4 spin klin series
Spin model
Parisi spin glass
Proton spin crisis
Spin off software
Spin operating system
Electric dipole spin resonance
Numeros cuanticos del oxigeno n l m s
Si unit of angular acceleration
Spin 1/3