The Pure Rotational Spectrum of Pivaloyl Chloride CH
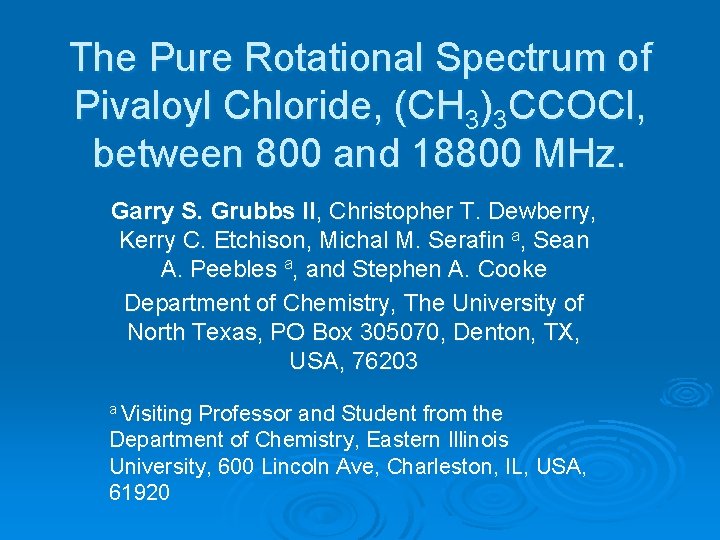
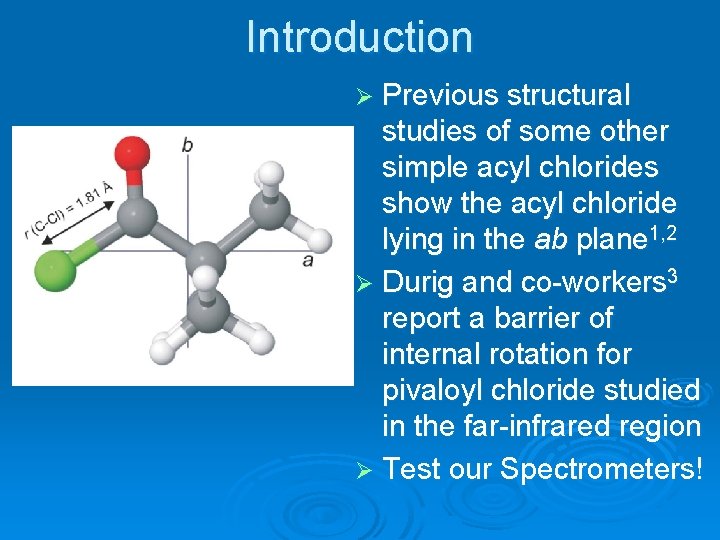
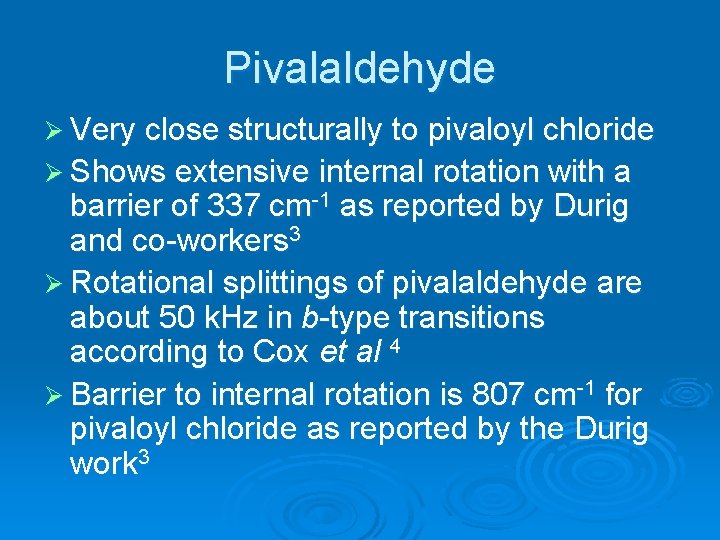
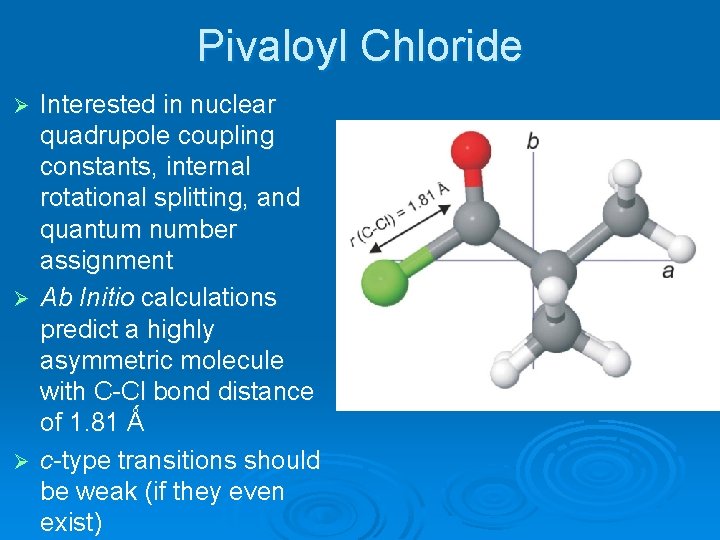
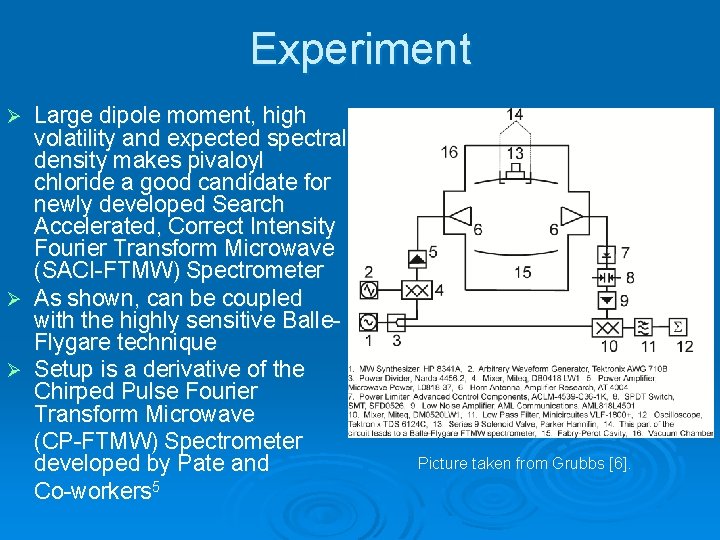
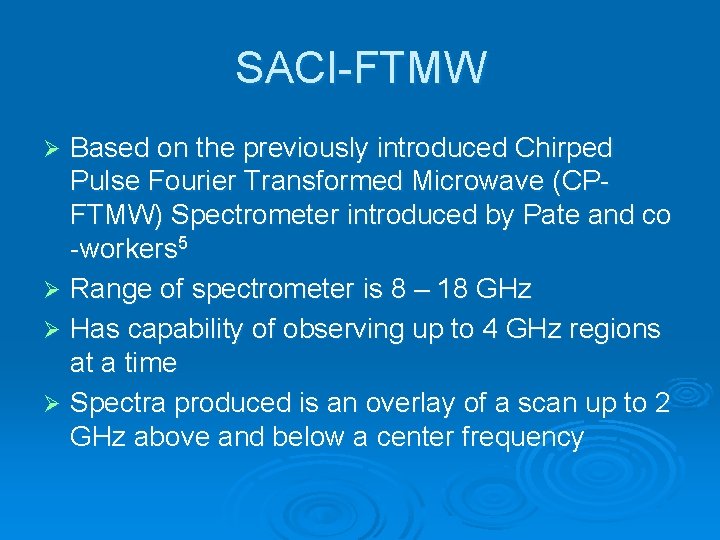
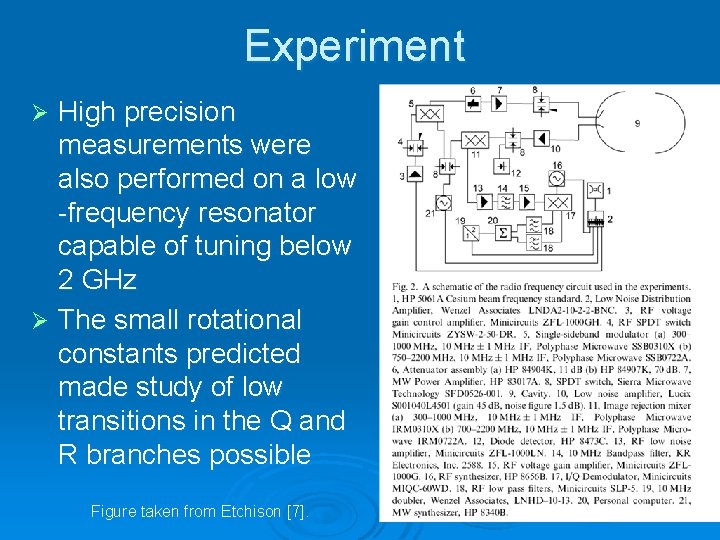
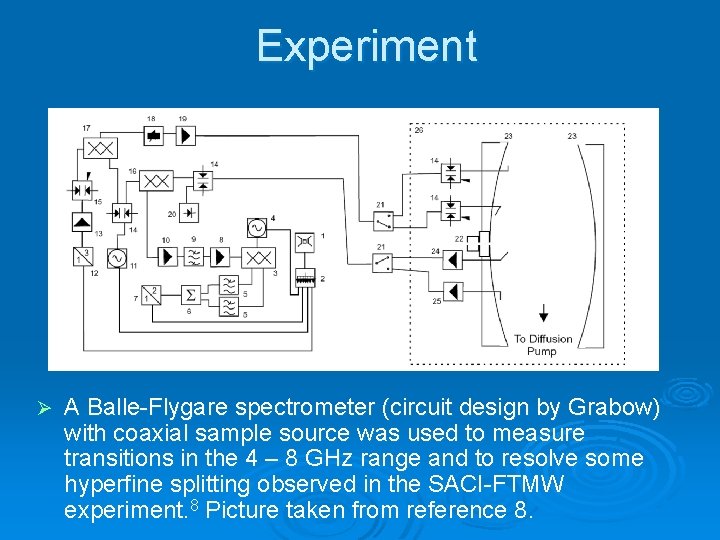
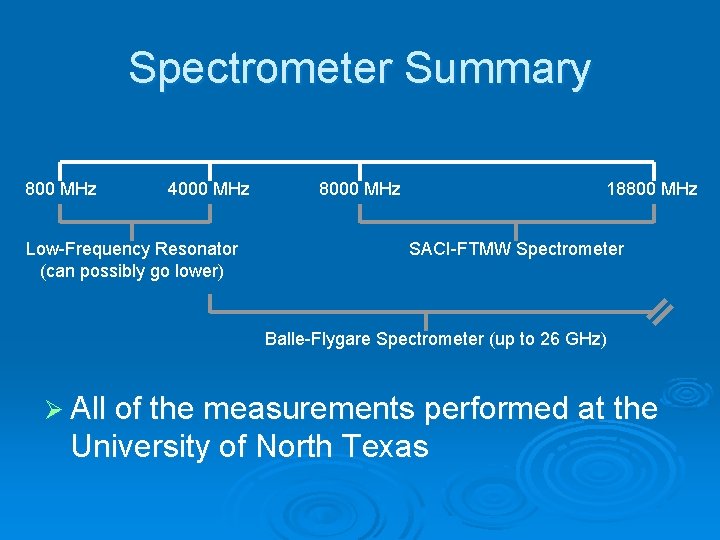
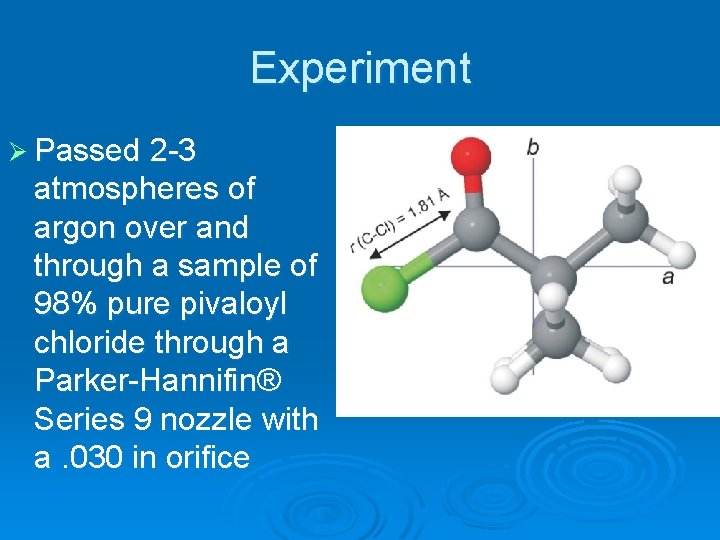
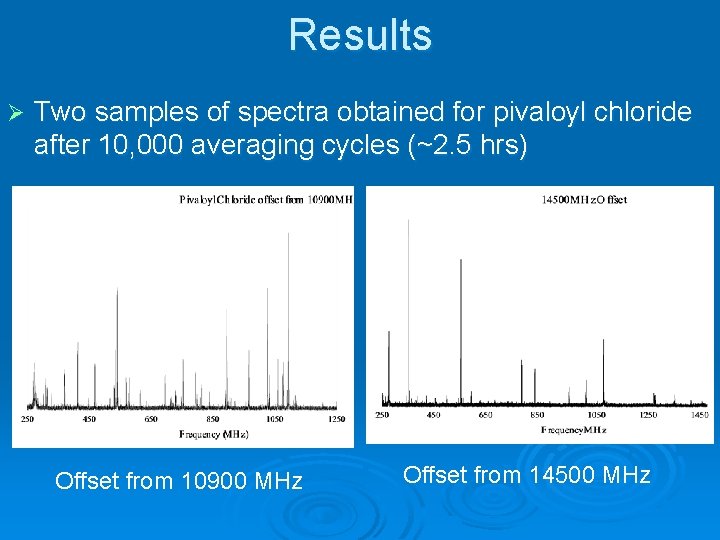
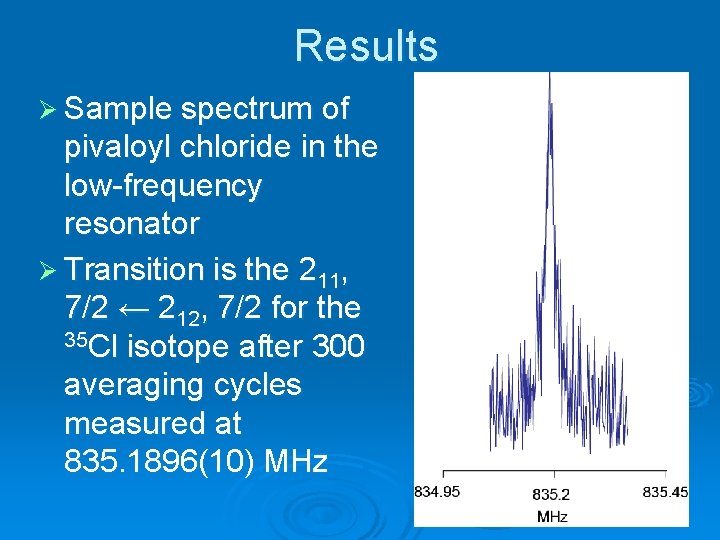
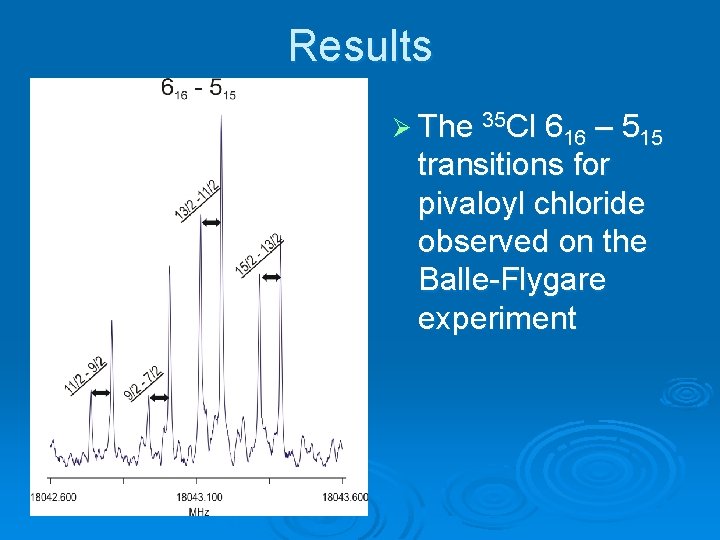
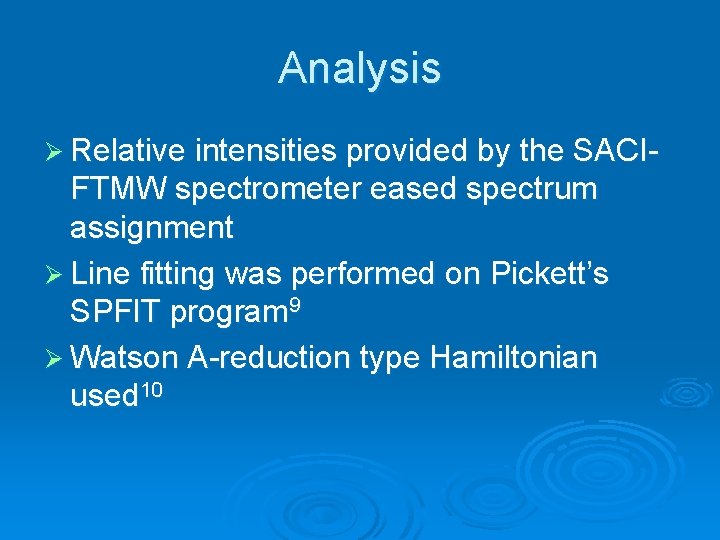
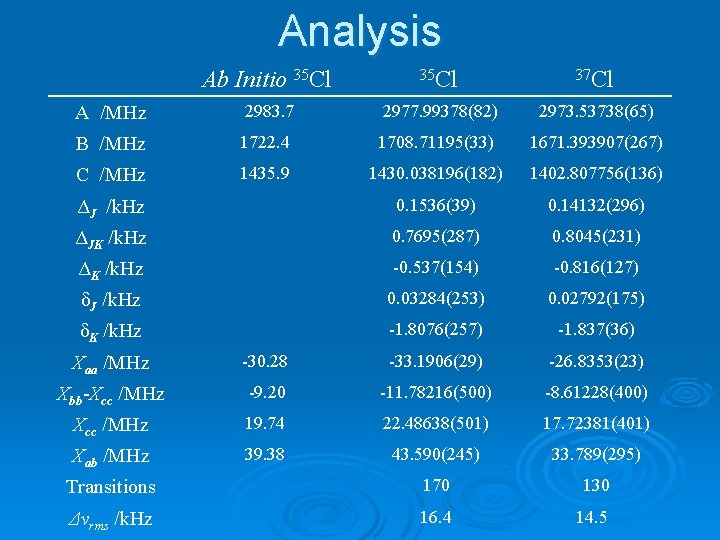
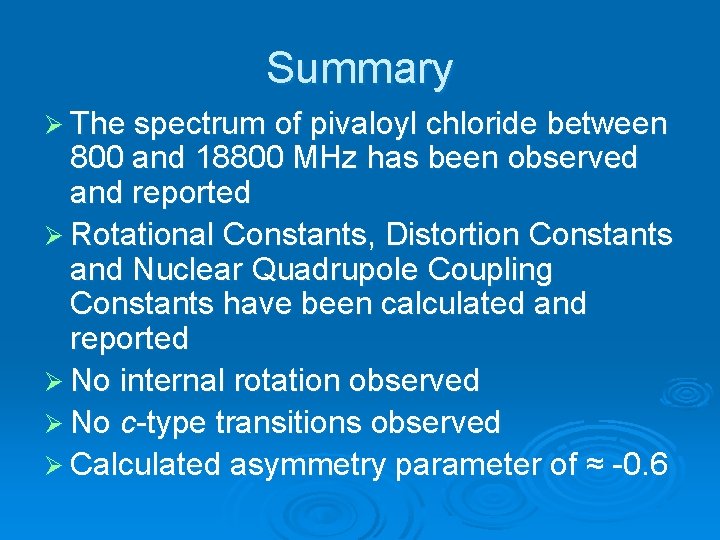
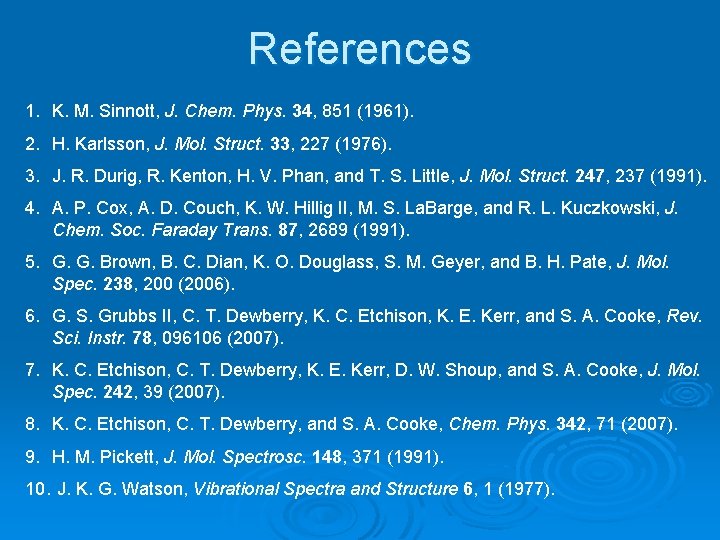
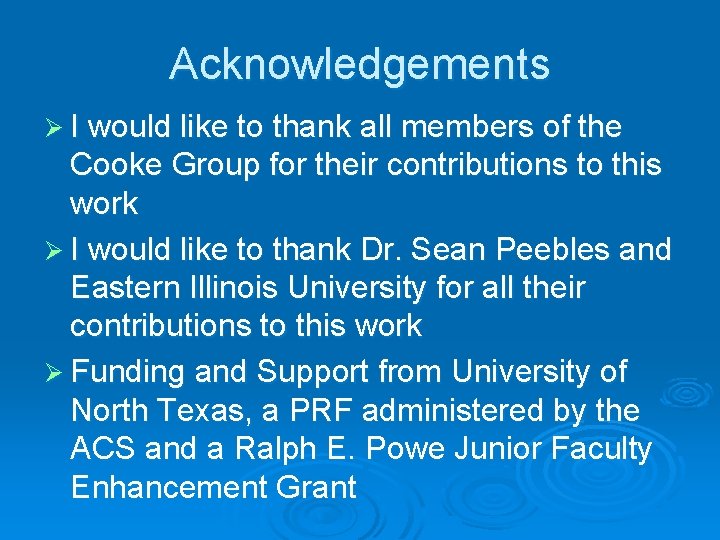
- Slides: 18
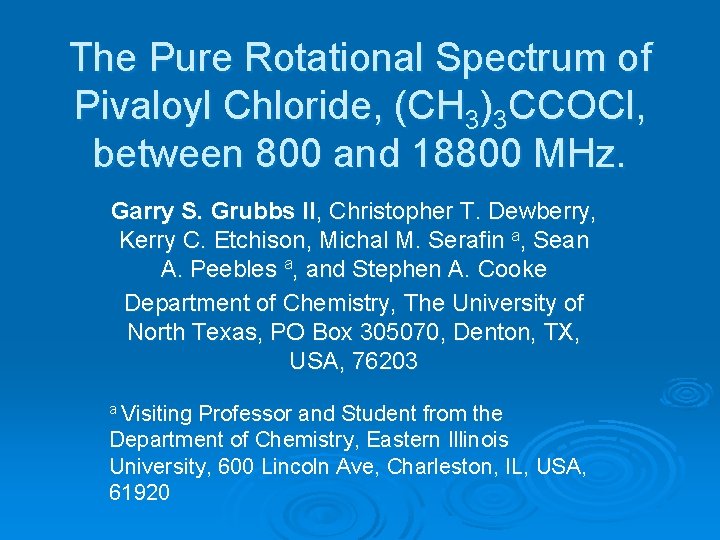
The Pure Rotational Spectrum of Pivaloyl Chloride, (CH 3)3 CCOCl, between 800 and 18800 MHz. Garry S. Grubbs II, Christopher T. Dewberry, Kerry C. Etchison, Michal M. Serafin a, Sean A. Peebles a, and Stephen A. Cooke Department of Chemistry, The University of North Texas, PO Box 305070, Denton, TX, USA, 76203 a Visiting Professor and Student from the Department of Chemistry, Eastern Illinois University, 600 Lincoln Ave, Charleston, IL, USA, 61920
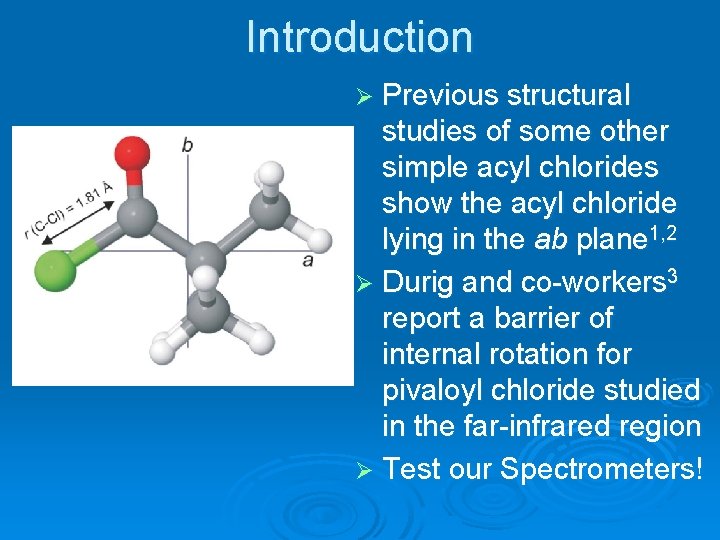
Introduction Ø Previous structural studies of some other simple acyl chlorides show the acyl chloride lying in the ab plane 1, 2 Ø Durig and co-workers 3 report a barrier of internal rotation for pivaloyl chloride studied in the far-infrared region Ø Test our Spectrometers!
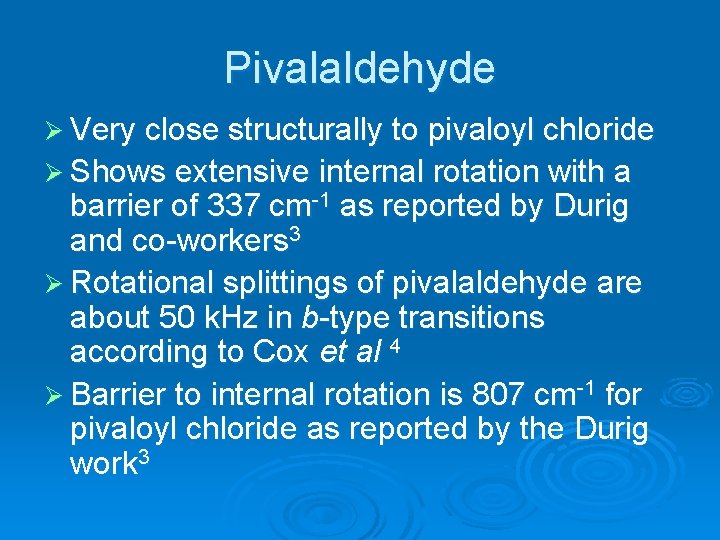
Pivalaldehyde Ø Very close structurally to pivaloyl chloride Ø Shows extensive internal rotation with a barrier of 337 cm-1 as reported by Durig and co-workers 3 Ø Rotational splittings of pivalaldehyde are about 50 k. Hz in b-type transitions according to Cox et al 4 Ø Barrier to internal rotation is 807 cm-1 for pivaloyl chloride as reported by the Durig work 3
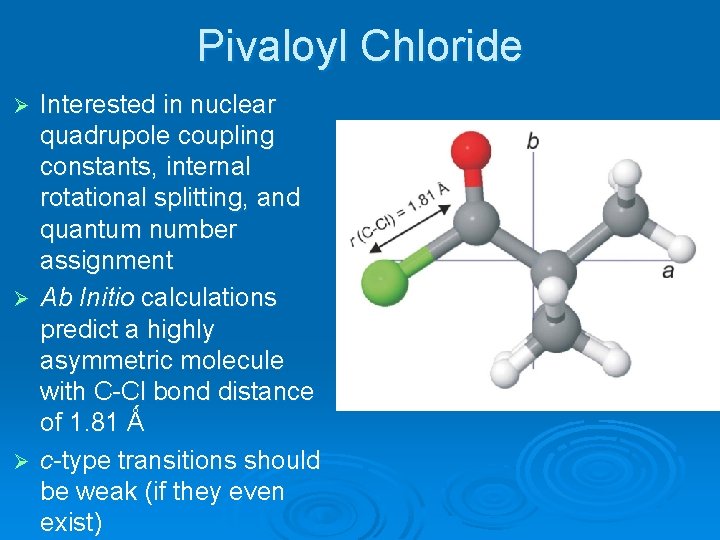
Pivaloyl Chloride Interested in nuclear quadrupole coupling constants, internal rotational splitting, and quantum number assignment Ø Ab Initio calculations predict a highly asymmetric molecule with C-Cl bond distance of 1. 81 Ǻ Ø c-type transitions should be weak (if they even exist) Ø
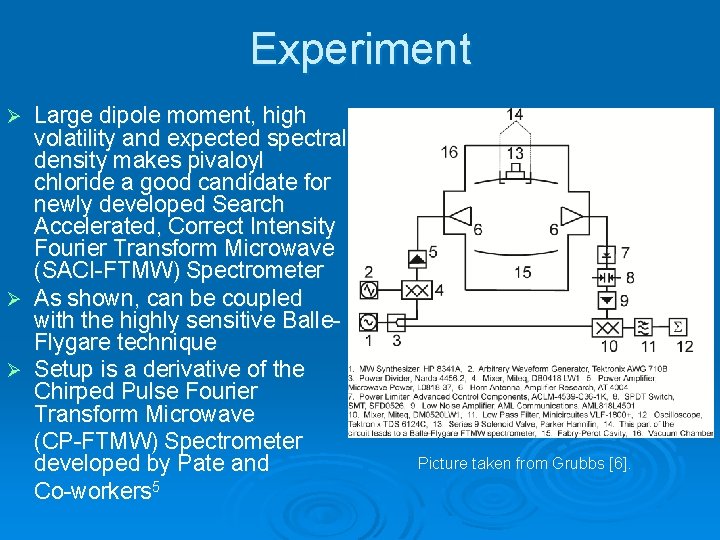
Experiment Large dipole moment, high volatility and expected spectral density makes pivaloyl chloride a good candidate for newly developed Search Accelerated, Correct Intensity Fourier Transform Microwave (SACI-FTMW) Spectrometer Ø As shown, can be coupled with the highly sensitive Balle. Flygare technique Ø Setup is a derivative of the Chirped Pulse Fourier Transform Microwave (CP-FTMW) Spectrometer developed by Pate and Co-workers 5 Ø Picture taken from Grubbs [6].
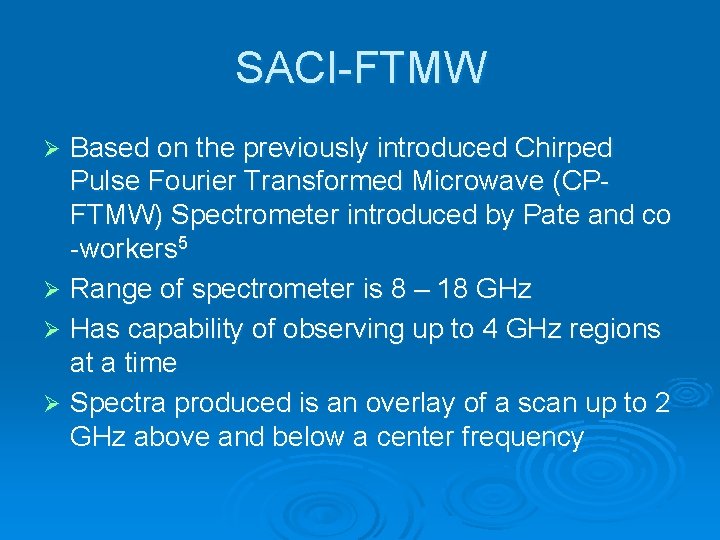
SACI-FTMW Based on the previously introduced Chirped Pulse Fourier Transformed Microwave (CPFTMW) Spectrometer introduced by Pate and co -workers 5 Ø Range of spectrometer is 8 – 18 GHz Ø Has capability of observing up to 4 GHz regions at a time Ø Spectra produced is an overlay of a scan up to 2 GHz above and below a center frequency Ø
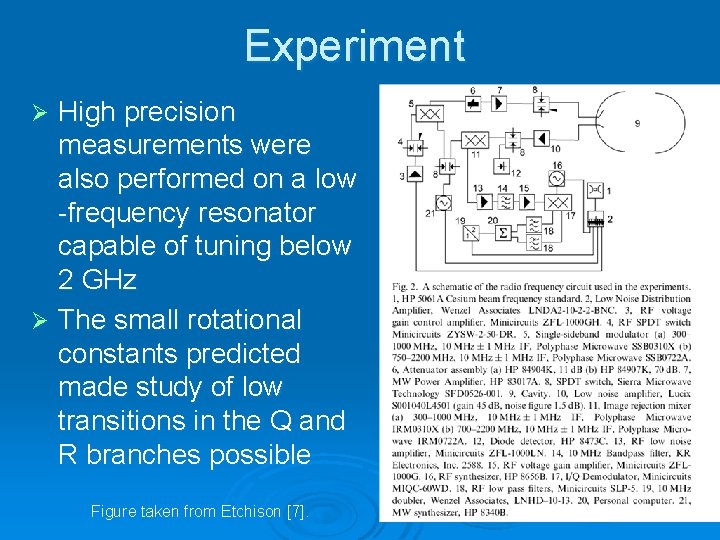
Experiment High precision measurements were also performed on a low -frequency resonator capable of tuning below 2 GHz Ø The small rotational constants predicted made study of low transitions in the Q and R branches possible Ø Figure taken from Etchison [7].
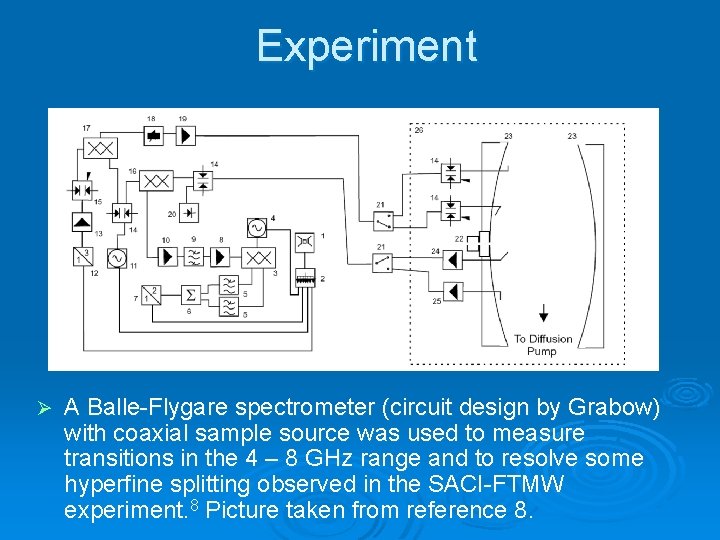
Experiment Ø A Balle-Flygare spectrometer (circuit design by Grabow) with coaxial sample source was used to measure transitions in the 4 – 8 GHz range and to resolve some hyperfine splitting observed in the SACI-FTMW experiment. 8 Picture taken from reference 8.
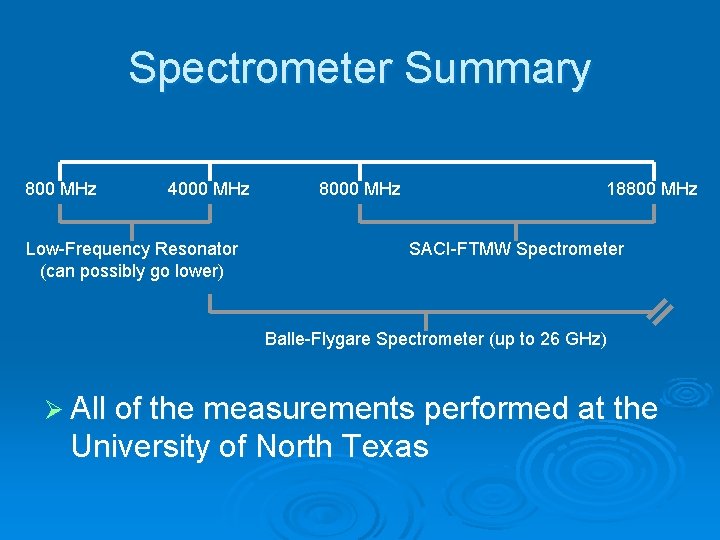
Spectrometer Summary 800 MHz 4000 MHz Low-Frequency Resonator (can possibly go lower) 8000 MHz 18800 MHz SACI-FTMW Spectrometer Balle-Flygare Spectrometer (up to 26 GHz) Ø All of the measurements performed at the University of North Texas
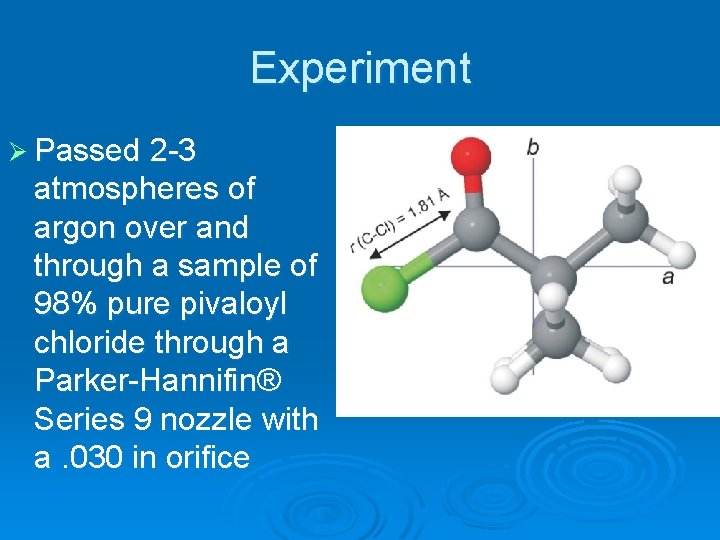
Experiment Ø Passed 2 -3 atmospheres of argon over and through a sample of 98% pure pivaloyl chloride through a Parker-Hannifin® Series 9 nozzle with a. 030 in orifice
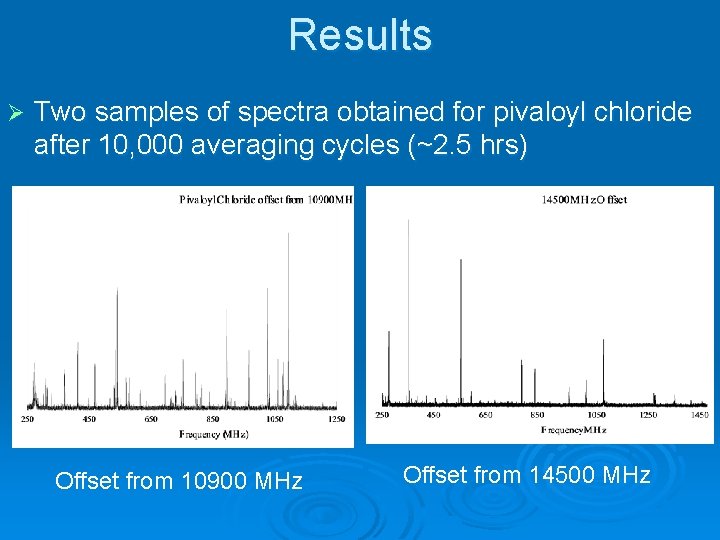
Results Ø Two samples of spectra obtained for pivaloyl chloride after 10, 000 averaging cycles (~2. 5 hrs) Offset from 10900 MHz Offset from 14500 MHz
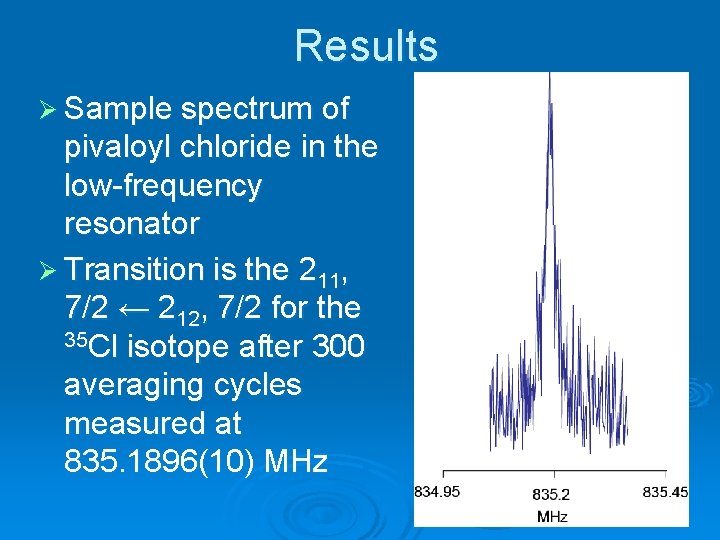
Results Ø Sample spectrum of pivaloyl chloride in the low-frequency resonator Ø Transition is the 211, 7/2 ← 212, 7/2 for the 35 Cl isotope after 300 averaging cycles measured at 835. 1896(10) MHz
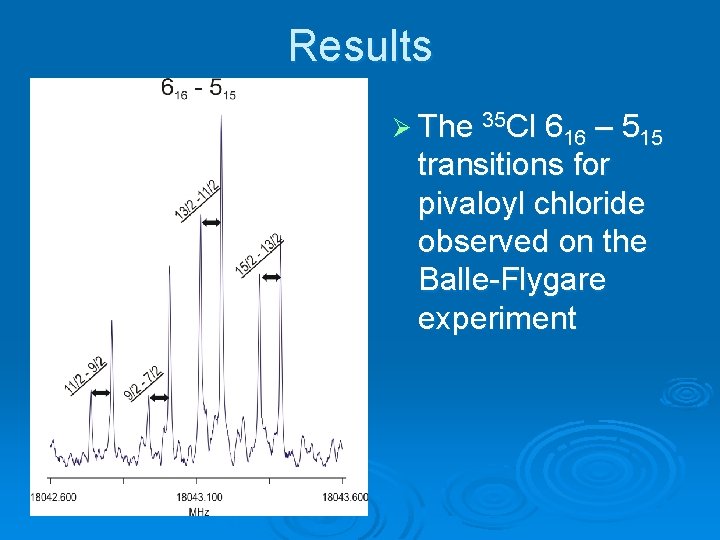
Results Ø The 35 Cl 616 – 515 transitions for pivaloyl chloride observed on the Balle-Flygare experiment
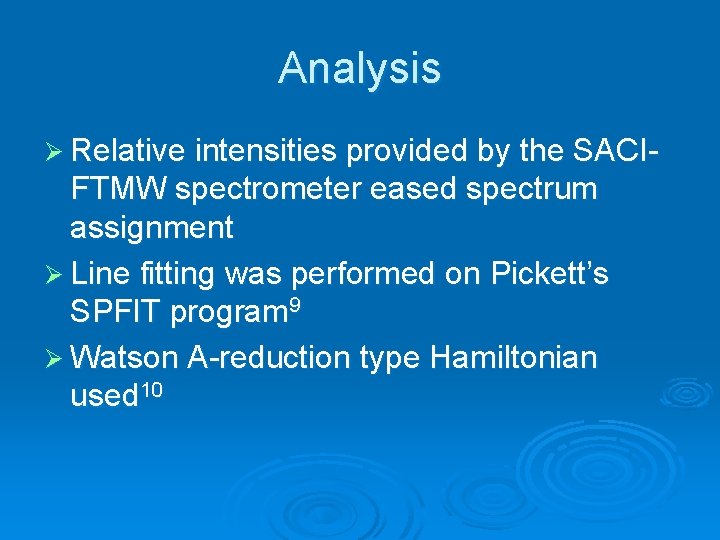
Analysis Ø Relative intensities provided by the SACI- FTMW spectrometer eased spectrum assignment Ø Line fitting was performed on Pickett’s SPFIT program 9 Ø Watson A-reduction type Hamiltonian used 10
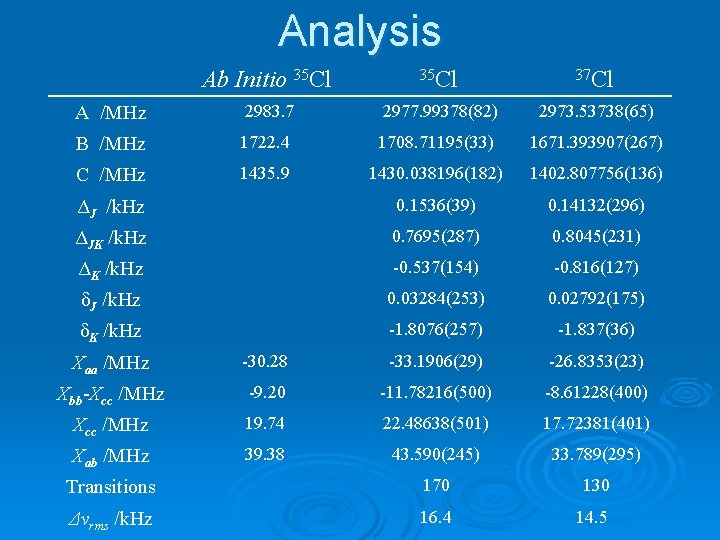
Analysis Ab Initio 35 Cl 37 Cl A /MHz 2983. 7 2977. 99378(82) 2973. 53738(65) B /MHz 1722. 4 1708. 71195(33) 1671. 393907(267) C /MHz 1435. 9 ΔJ /k. Hz 0. 1536(39) 0. 14132(296) ΔJK /k. Hz 0. 7695(287) 0. 8045(231) ΔK /k. Hz -0. 537(154) -0. 816(127) δJ /k. Hz 0. 03284(253) 0. 02792(175) δK /k. Hz -1. 8076(257) -1. 837(36) Χaa /MHz -30. 28 -33. 1906(29) -26. 8353(23) Χbb-Χcc /MHz -9. 20 -11. 78216(500) -8. 61228(400) Χcc /MHz 19. 74 22. 48638(501) 17. 72381(401) Χab /MHz 39. 38 43. 590(245) 33. 789(295) Transitions 170 130 Δνrms /k. Hz 16. 4 14. 5 1430. 038196(182) 1402. 807756(136)
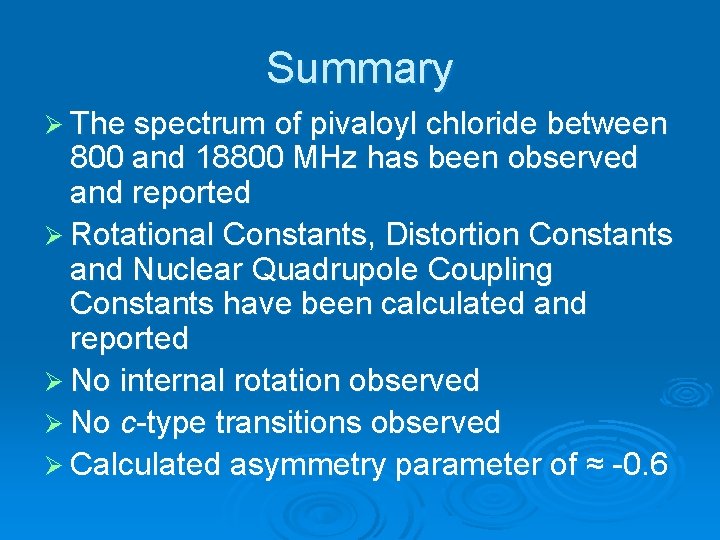
Summary Ø The spectrum of pivaloyl chloride between 800 and 18800 MHz has been observed and reported Ø Rotational Constants, Distortion Constants and Nuclear Quadrupole Coupling Constants have been calculated and reported Ø No internal rotation observed Ø No c-type transitions observed Ø Calculated asymmetry parameter of ≈ -0. 6
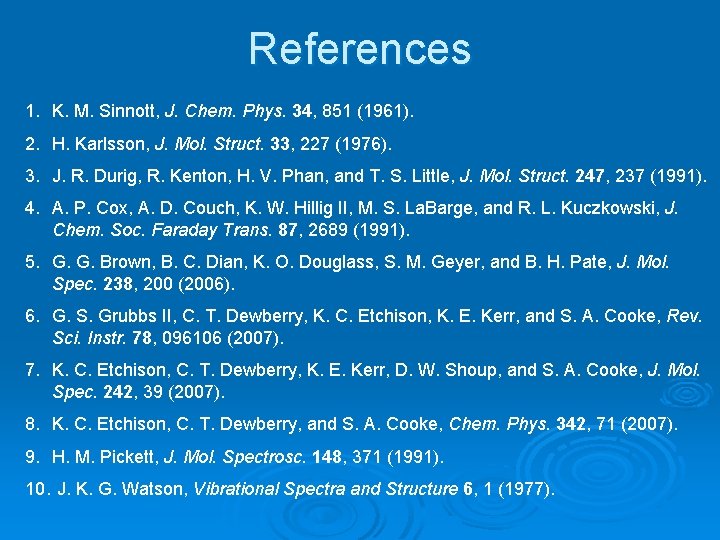
References 1. K. M. Sinnott, J. Chem. Phys. 34, 851 (1961). 2. H. Karlsson, J. Mol. Struct. 33, 227 (1976). 3. J. R. Durig, R. Kenton, H. V. Phan, and T. S. Little, J. Mol. Struct. 247, 237 (1991). 4. A. P. Cox, A. D. Couch, K. W. Hillig II, M. S. La. Barge, and R. L. Kuczkowski, J. Chem. Soc. Faraday Trans. 87, 2689 (1991). 5. G. G. Brown, B. C. Dian, K. O. Douglass, S. M. Geyer, and B. H. Pate, J. Mol. Spec. 238, 200 (2006). 6. G. S. Grubbs II, C. T. Dewberry, K. C. Etchison, K. E. Kerr, and S. A. Cooke, Rev. Sci. Instr. 78, 096106 (2007). 7. K. C. Etchison, C. T. Dewberry, K. E. Kerr, D. W. Shoup, and S. A. Cooke, J. Mol. Spec. 242, 39 (2007). 8. K. C. Etchison, C. T. Dewberry, and S. A. Cooke, Chem. Phys. 342, 71 (2007). 9. H. M. Pickett, J. Mol. Spectrosc. 148, 371 (1991). 10. J. K. G. Watson, Vibrational Spectra and Structure 6, 1 (1977).
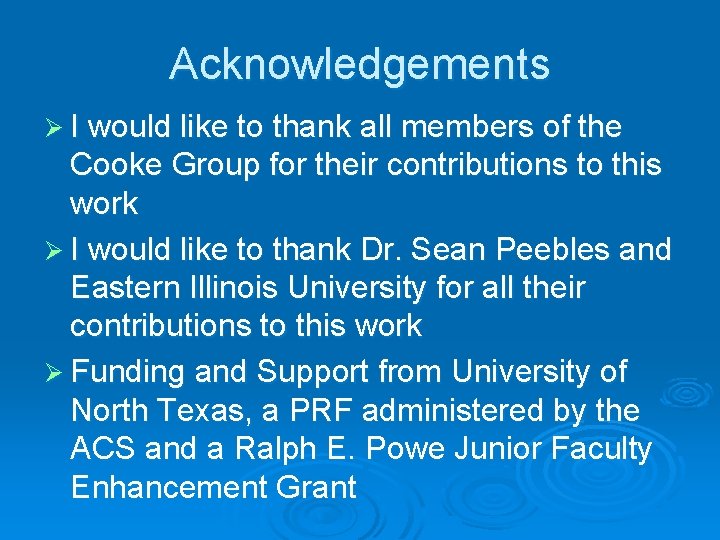
Acknowledgements Ø I would like to thank all members of the Cooke Group for their contributions to this work Ø I would like to thank Dr. Sean Peebles and Eastern Illinois University for all their contributions to this work Ø Funding and Support from University of North Texas, a PRF administered by the ACS and a Ralph E. Powe Junior Faculty Enhancement Grant
Rotational equilibrium and rotational dynamics
Second condition of equilibrium
Absortpion
Chromium and copper electron configuration
Pure spectrum of light
Thang điểm glasgow
đại từ thay thế
Vẽ hình chiếu đứng bằng cạnh của vật thể
Quá trình desamine hóa có thể tạo ra
Công thức tính độ biến thiên đông lượng
Thế nào là mạng điện lắp đặt kiểu nổi
Sự nuôi và dạy con của hổ
Các châu lục và đại dương trên thế giới
Dot
Biện pháp chống mỏi cơ
Bổ thể
Phản ứng thế ankan
Thiếu nhi thế giới liên hoan
Chúa sống lại