Low Area and Low Power TimetoDigital Converter for
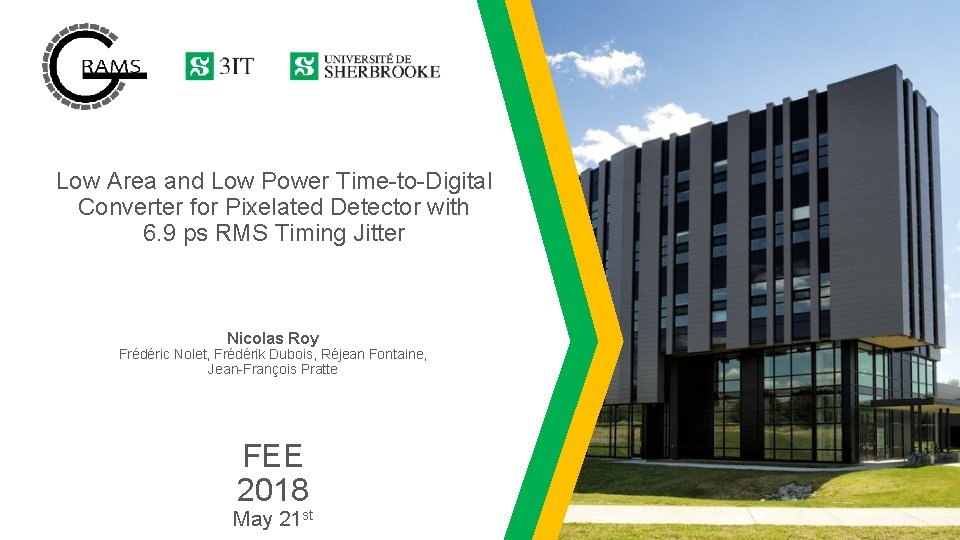
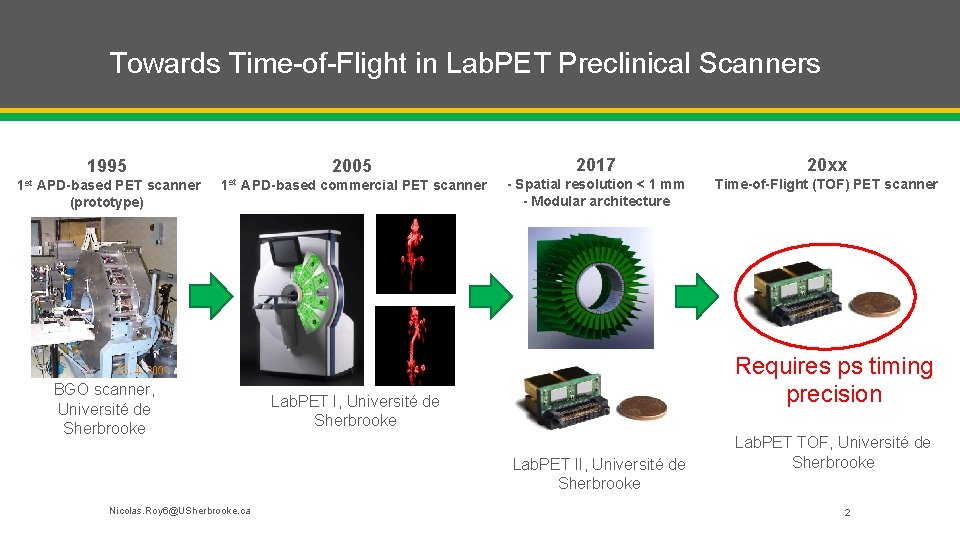
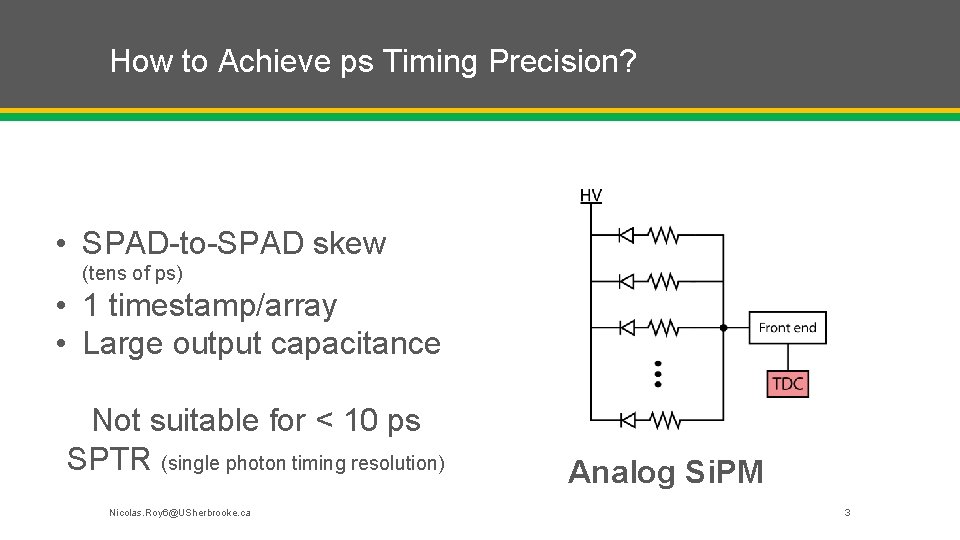
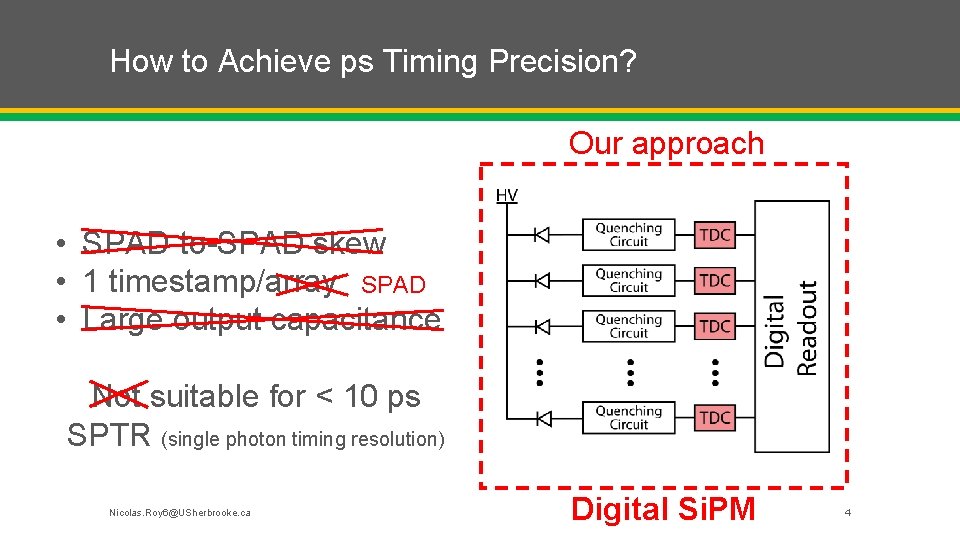
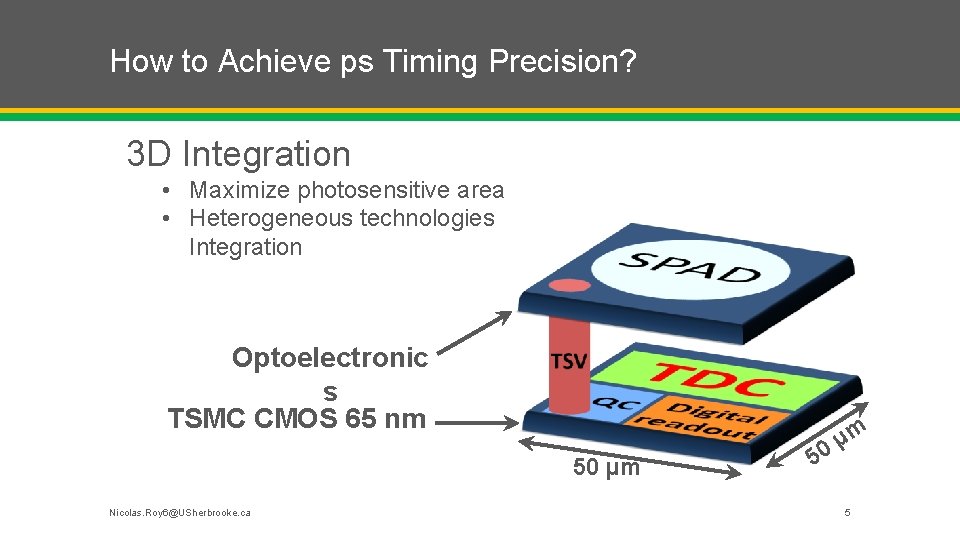
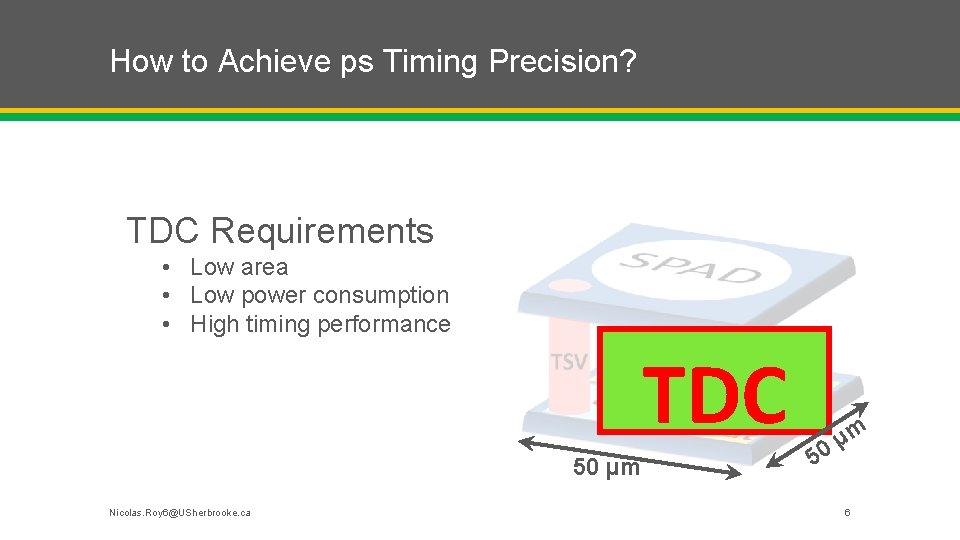
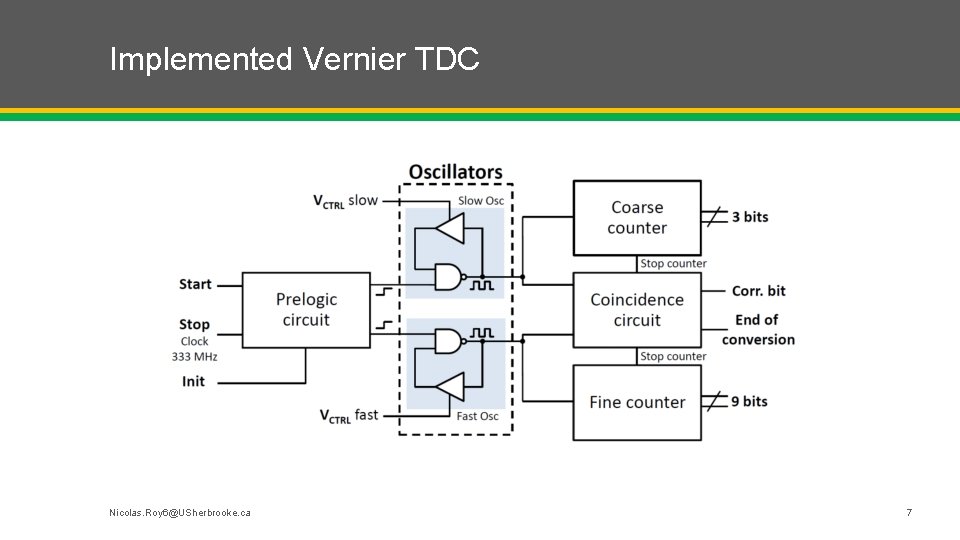
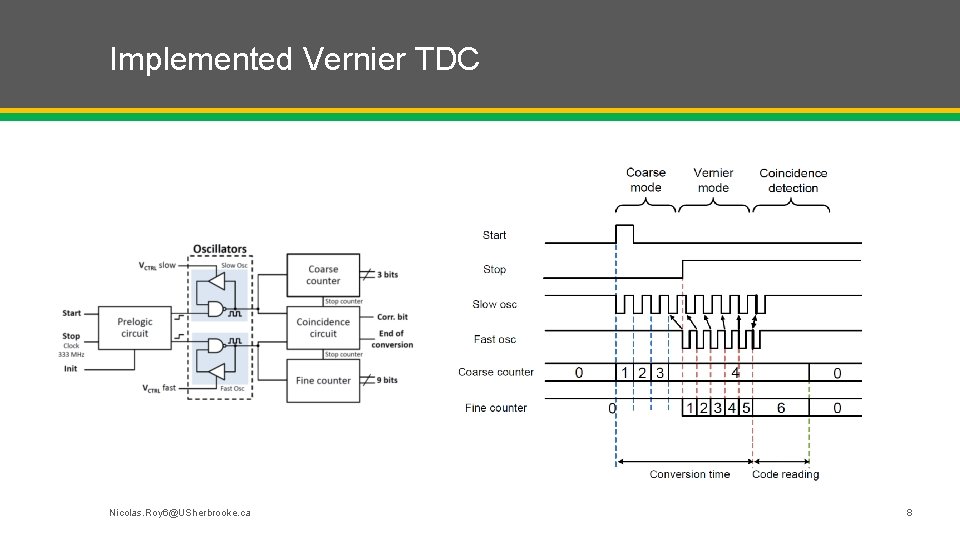
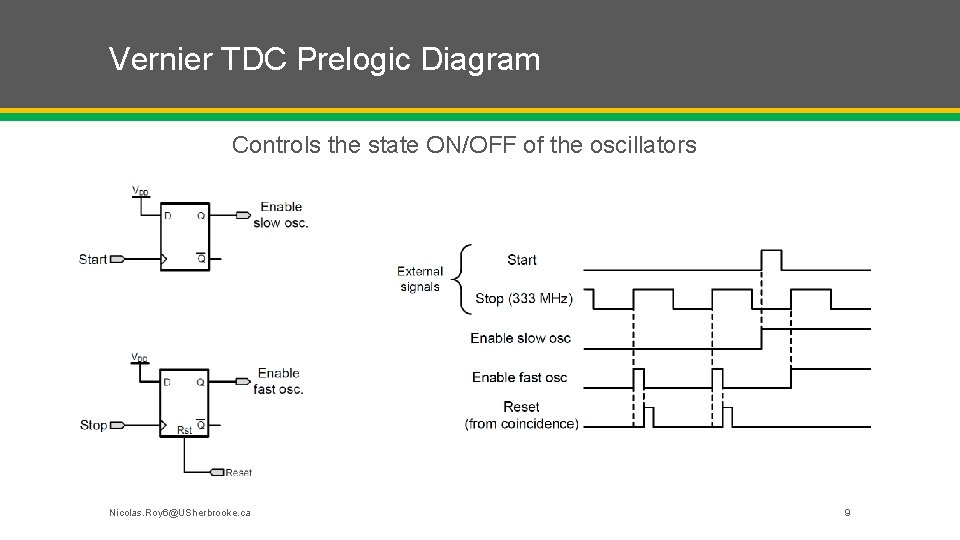
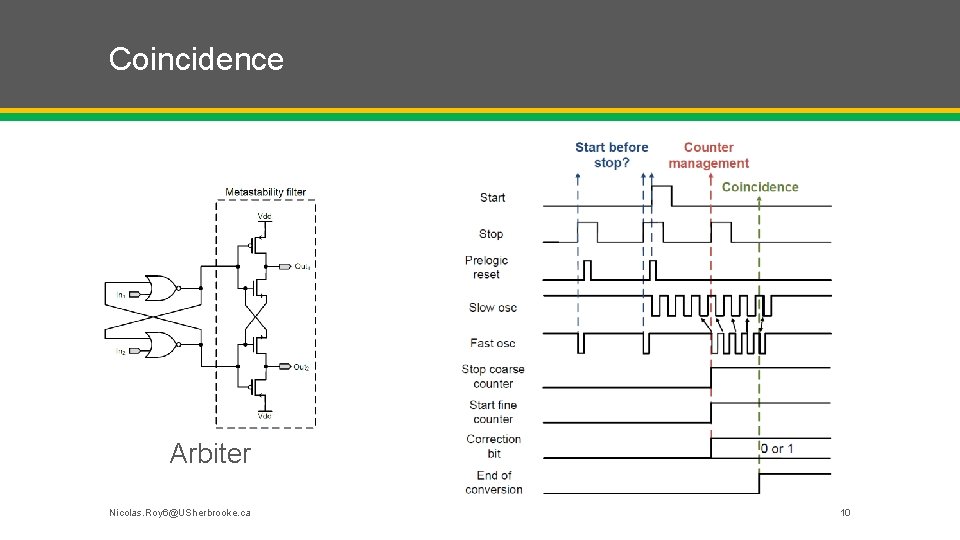
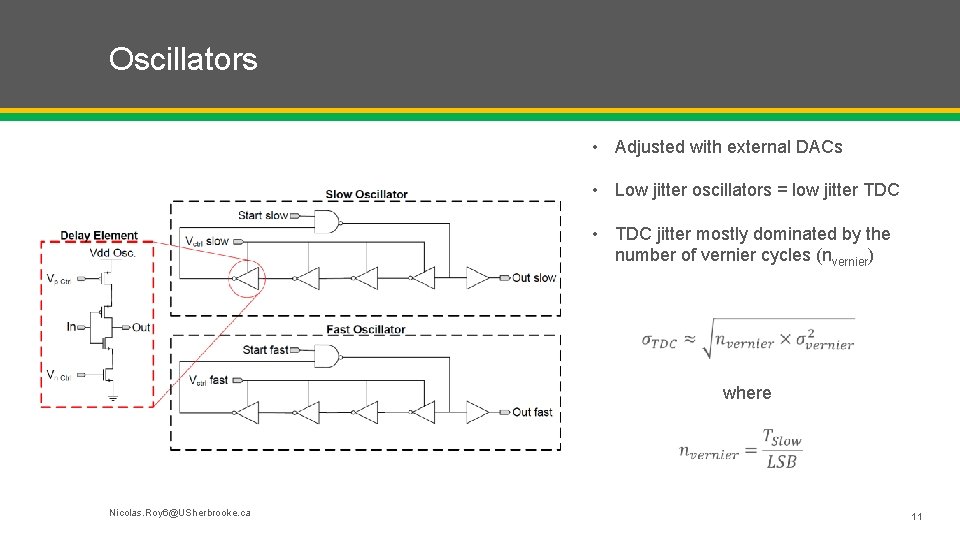
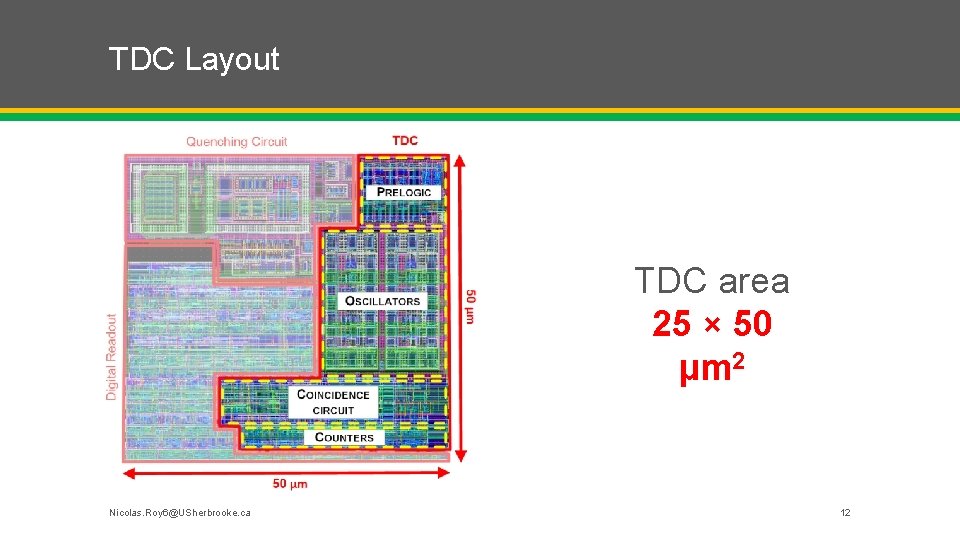
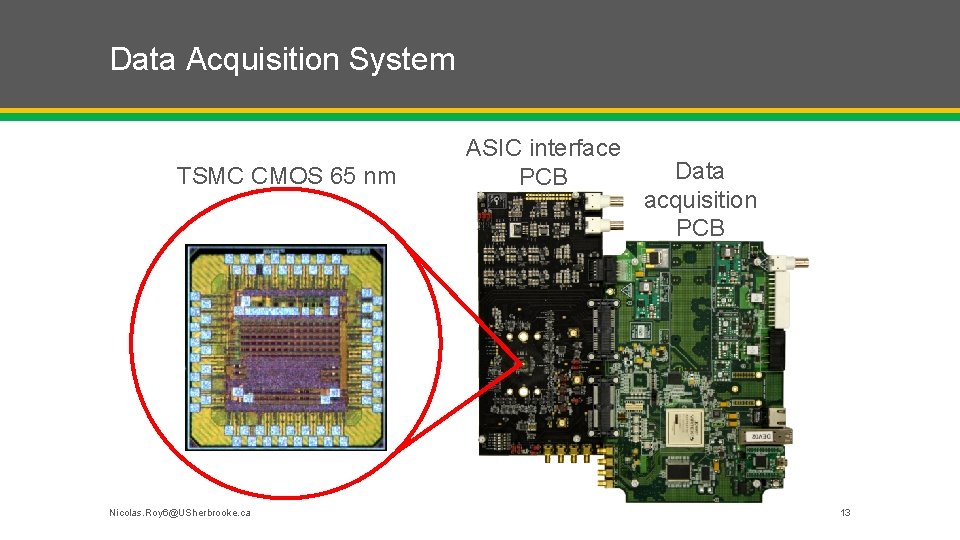
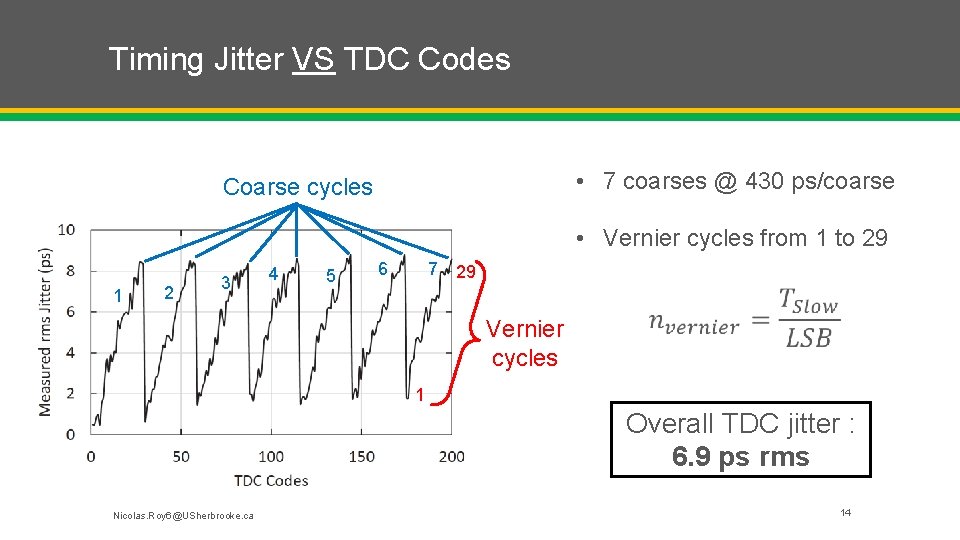
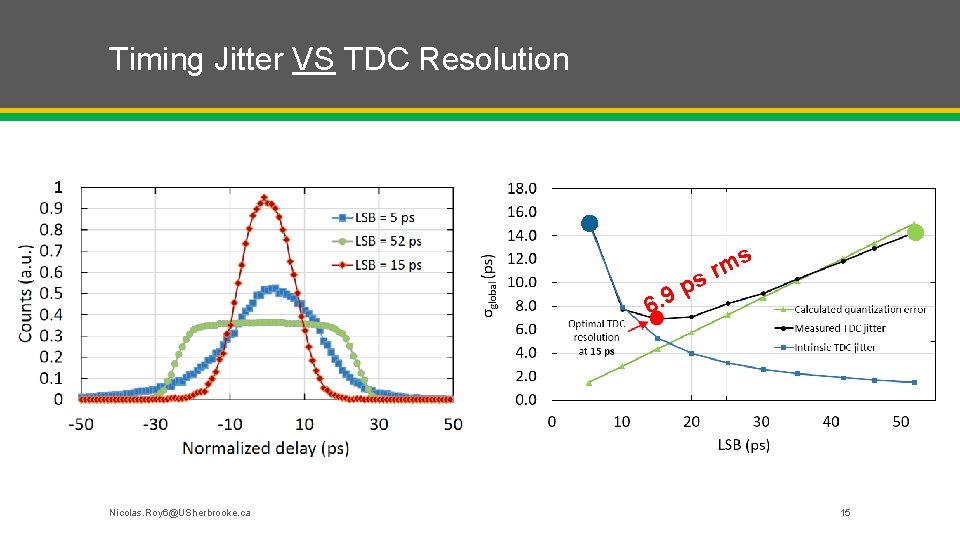
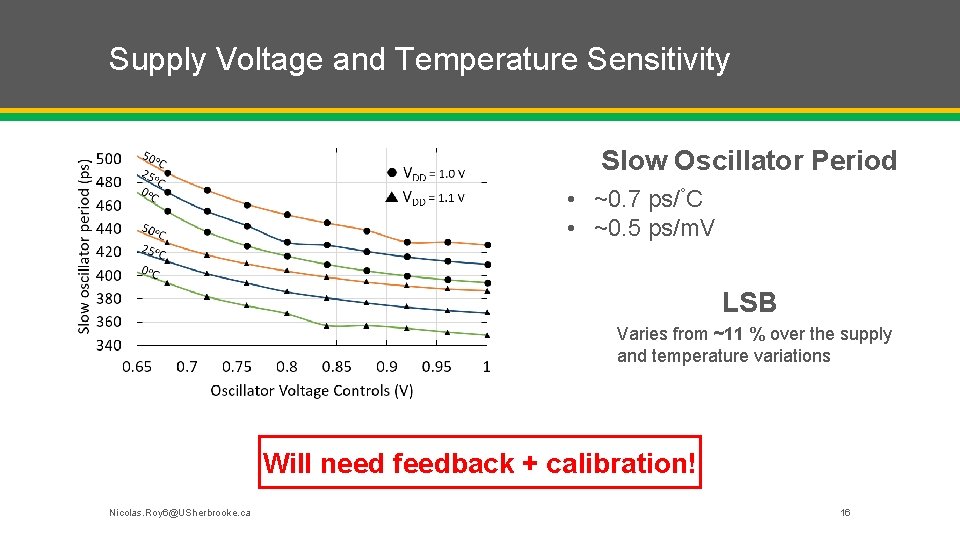
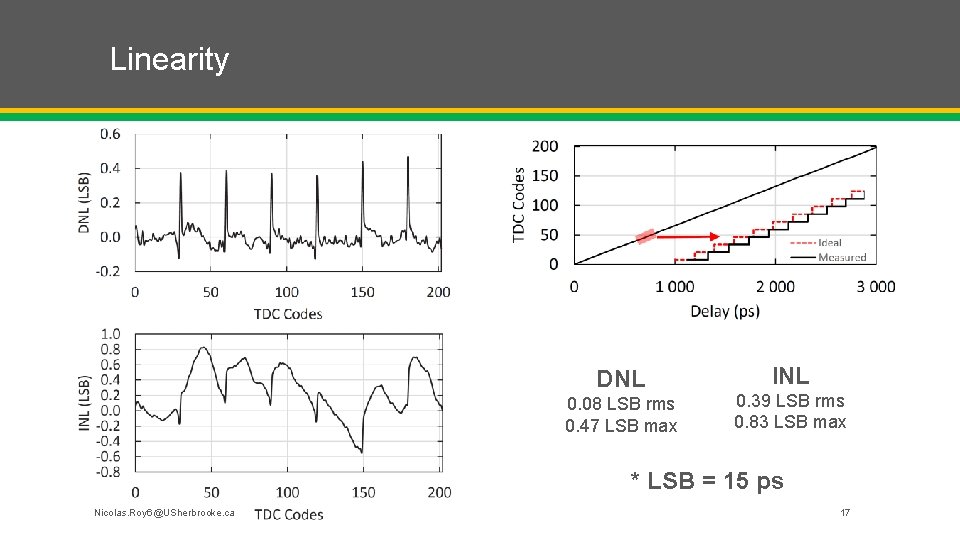
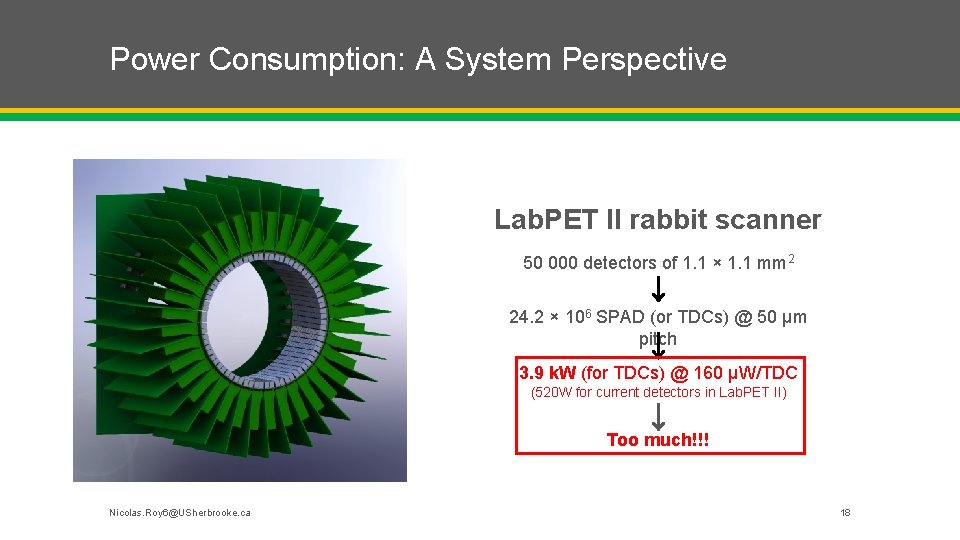
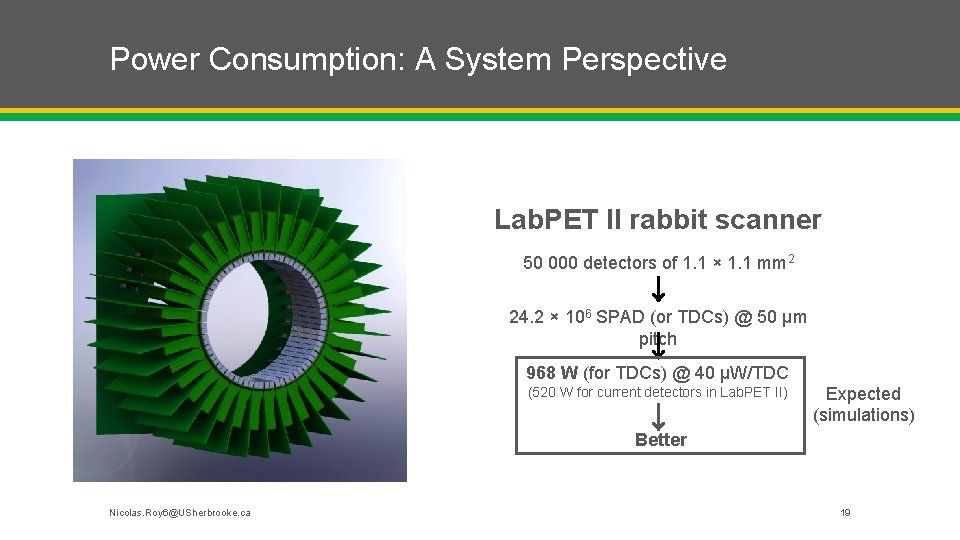
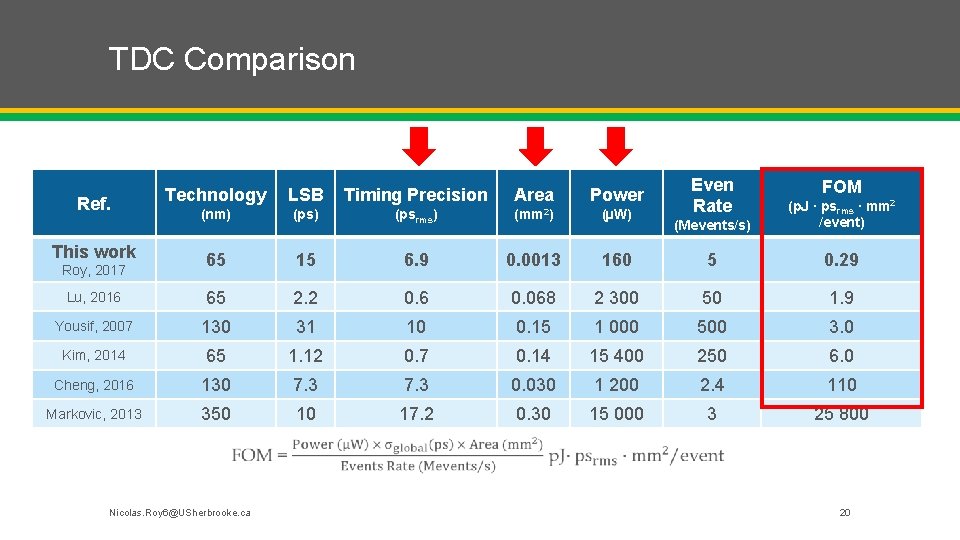
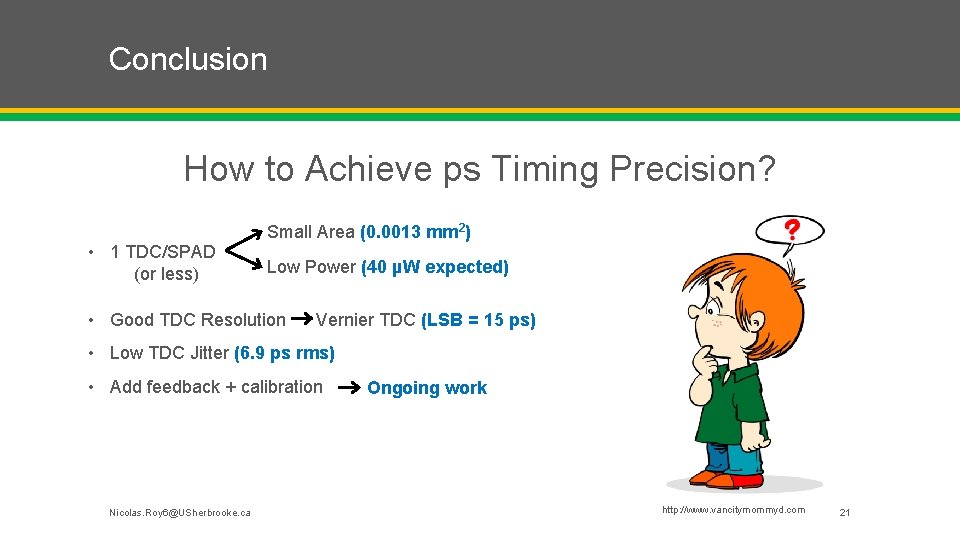
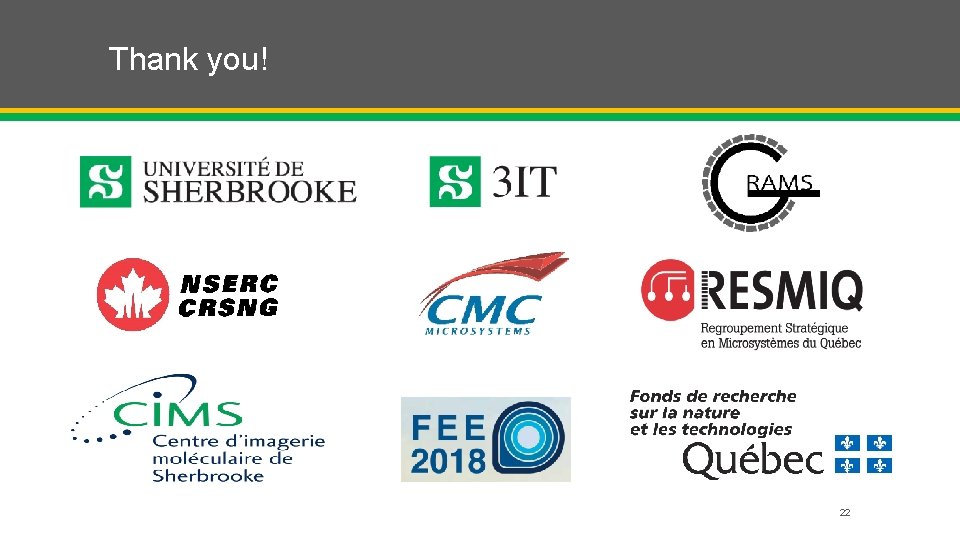
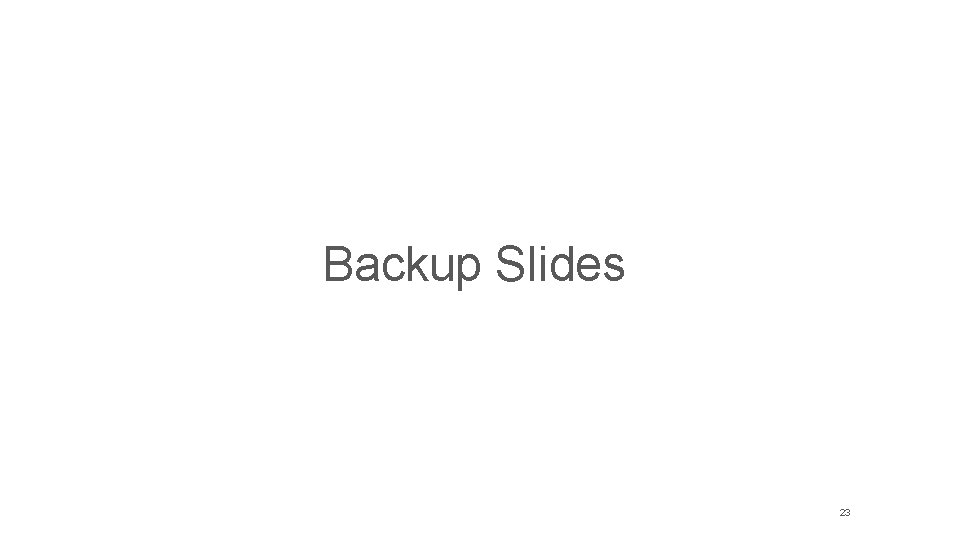
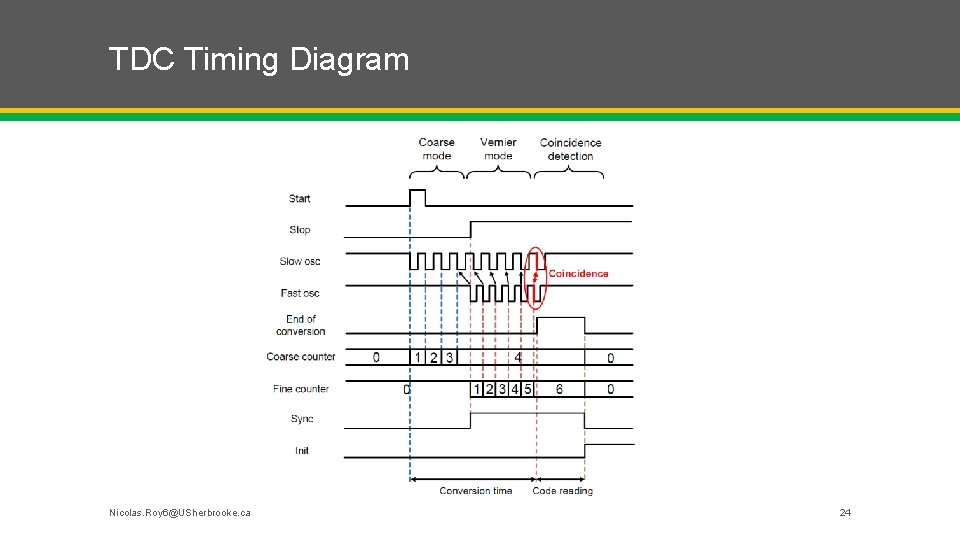
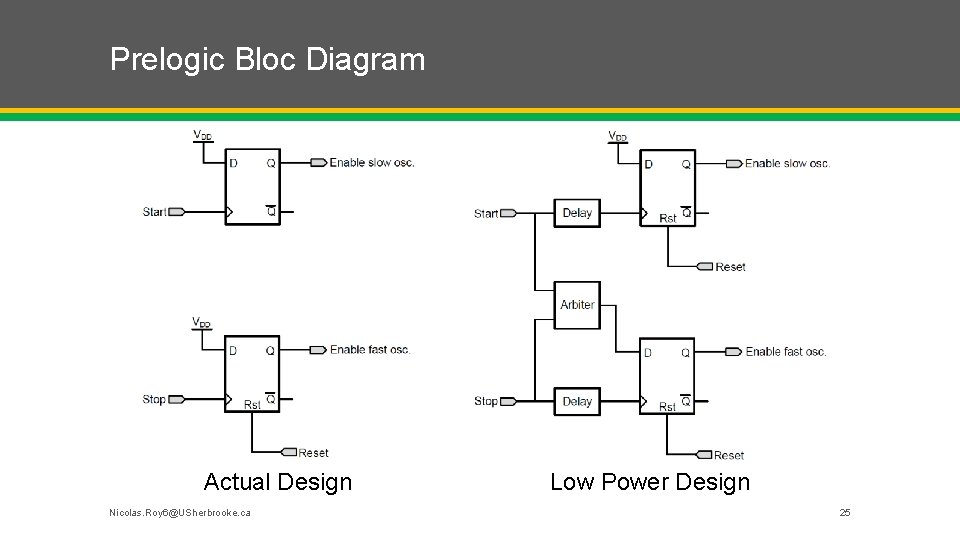
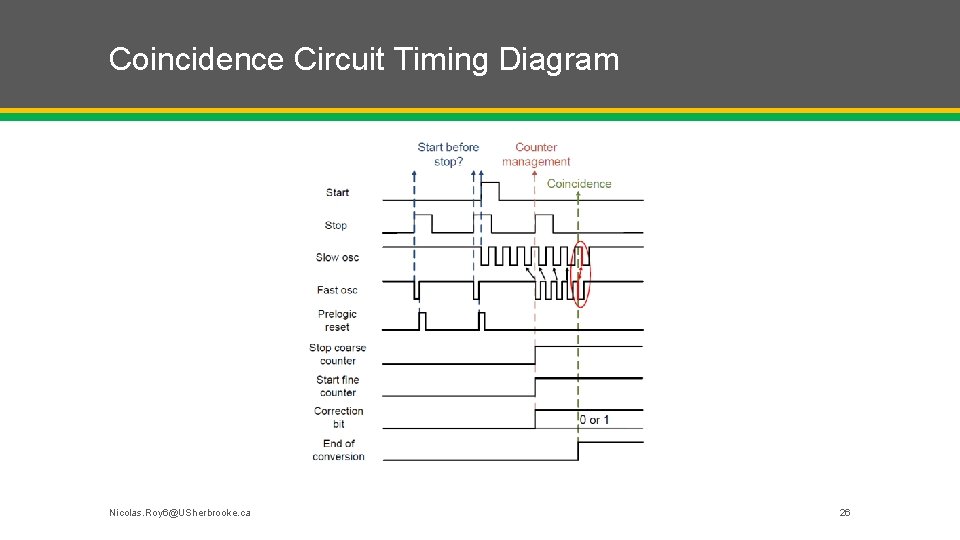
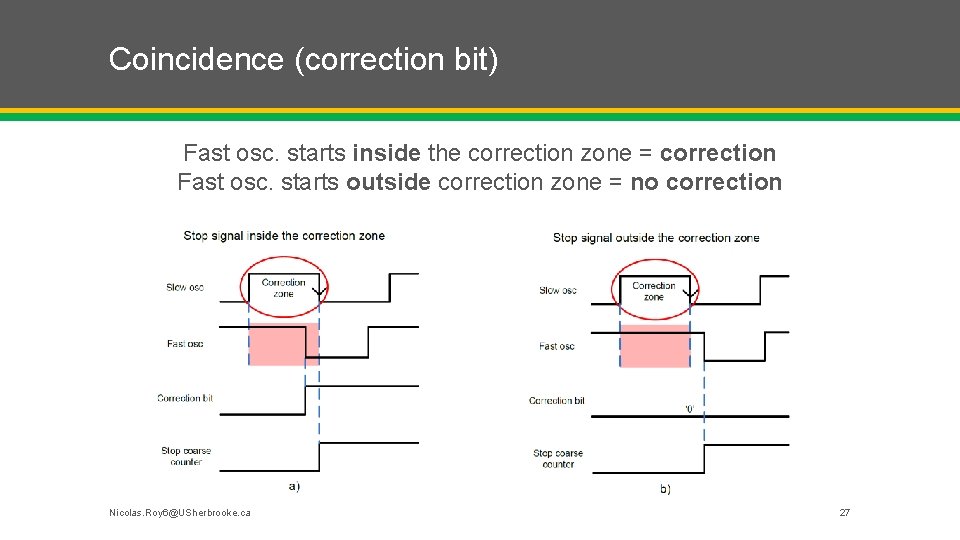
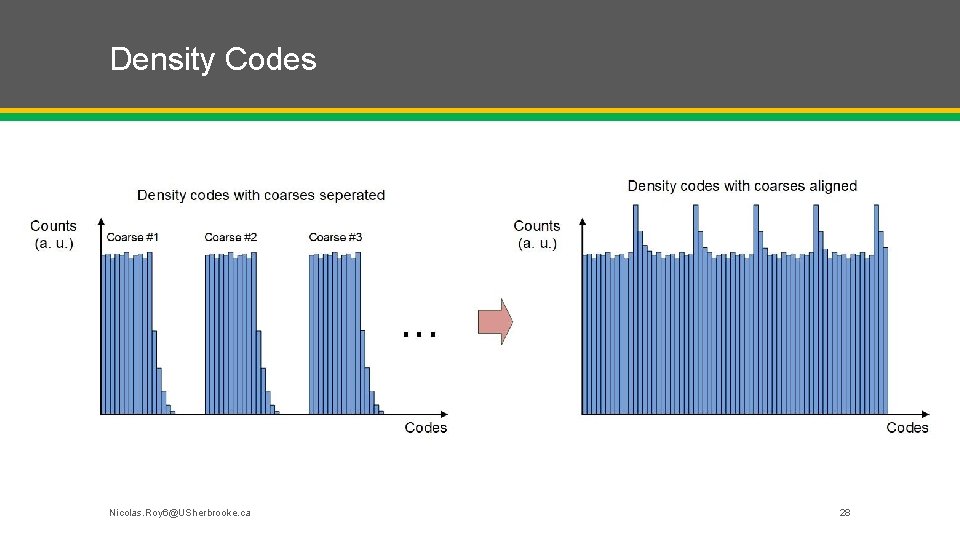
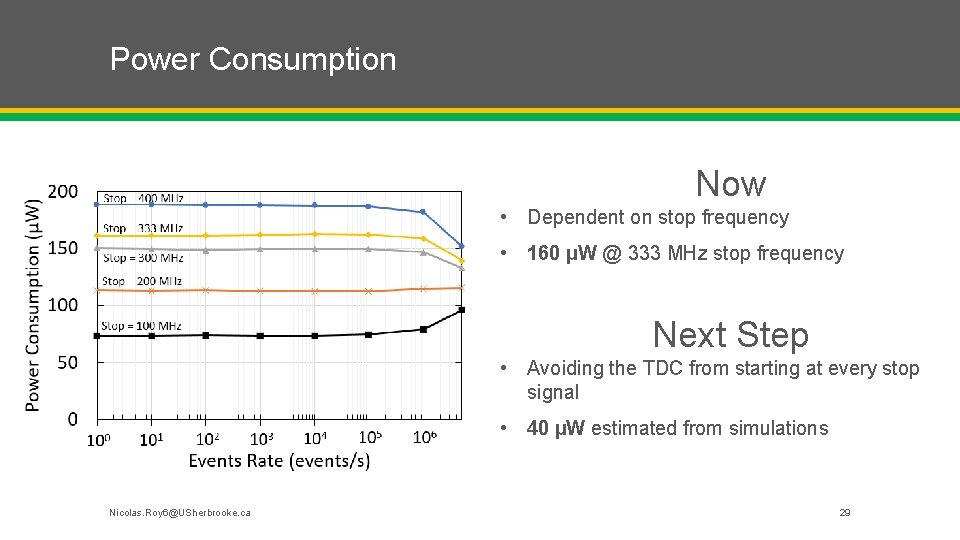
- Slides: 29
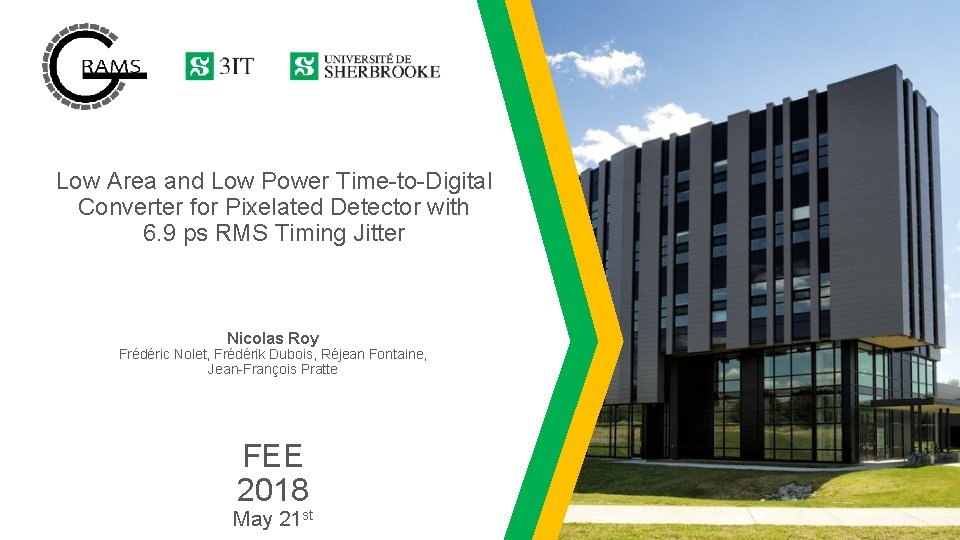
Low Area and Low Power Time-to-Digital Converter for Pixelated Detector with 6. 9 ps RMS Timing Jitter Nicolas Roy Frédéric Nolet, Frédérik Dubois, Réjean Fontaine, Jean-François Pratte FEE 2018 May 21 st
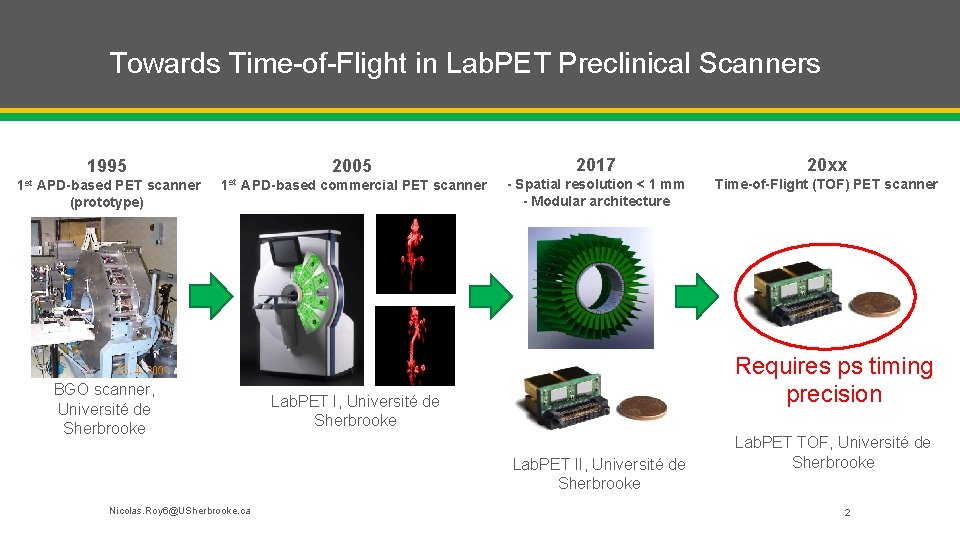
Towards Time-of-Flight in Lab. PET Preclinical Scanners 1995 2005 2017 20 xx 1 st APD-based PET scanner (prototype) 1 st APD-based commercial PET scanner - Spatial resolution < 1 mm - Modular architecture Time-of-Flight (TOF) PET scanner BGO scanner, Université de Sherbrooke Requires ps timing precision Lab. PET I, Université de Sherbrooke Lab. PET II, Université de Sherbrooke Nicolas. Roy 6@USherbrooke. ca Lab. PET TOF, Université de Sherbrooke 2
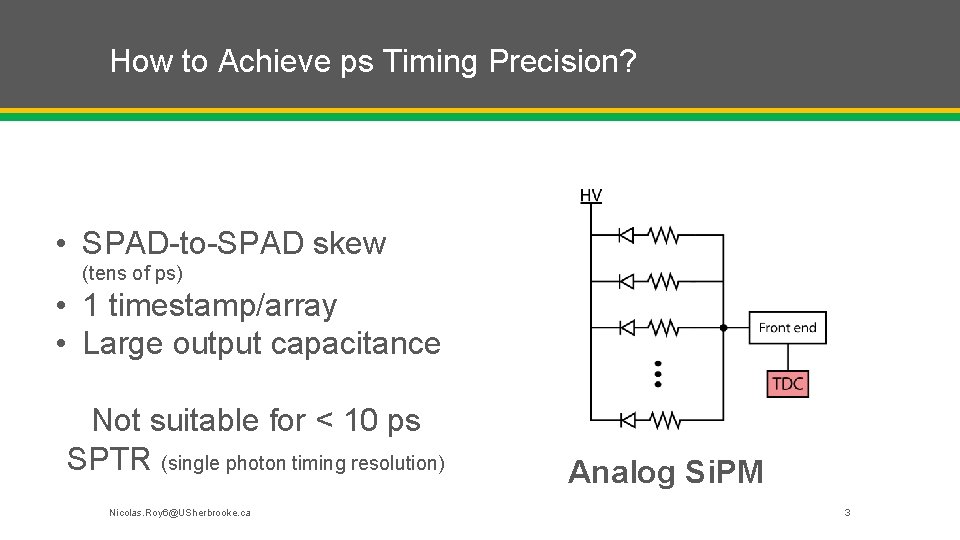
How to Achieve ps Timing Precision? • SPAD-to-SPAD skew (tens of ps) • 1 timestamp/array • Large output capacitance Not suitable for < 10 ps SPTR (single photon timing resolution) Nicolas. Roy 6@USherbrooke. ca Analog Si. PM 3
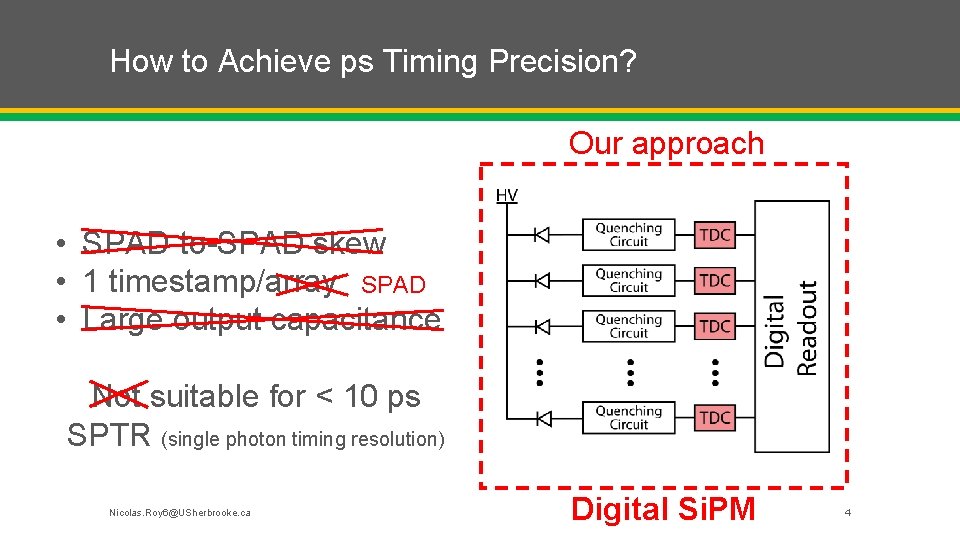
How to Achieve ps Timing Precision? Our approach • SPAD-to-SPAD skew • 1 timestamp/array SPAD • Large output capacitance Not suitable for < 10 ps SPTR (single photon timing resolution) Nicolas. Roy 6@USherbrooke. ca Digital Si. PM 4
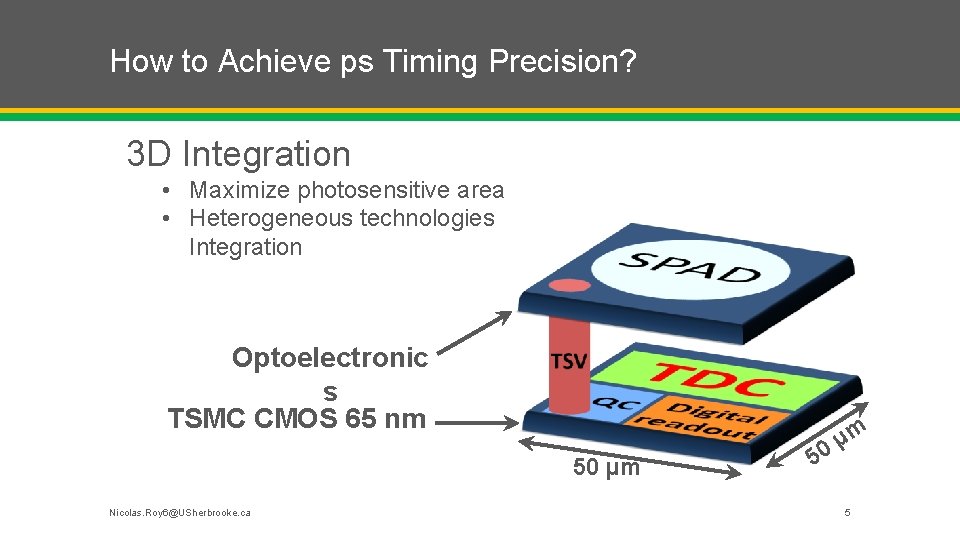
How to Achieve ps Timing Precision? 3 D Integration • Maximize photosensitive area • Heterogeneous technologies Integration Optoelectronic s TSMC CMOS 65 nm 50 µm Nicolas. Roy 6@USherbrooke. ca m µ 0 5 5
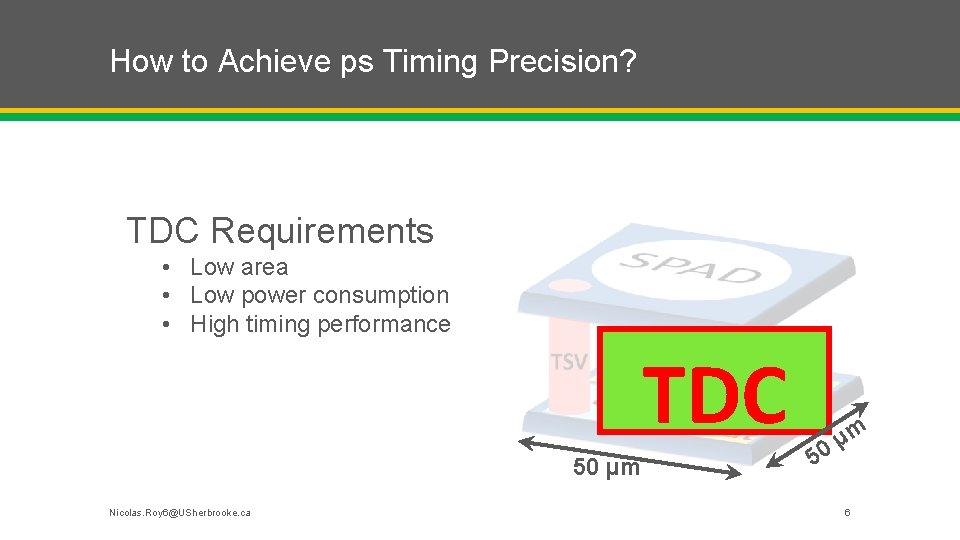
How to Achieve ps Timing Precision? TDC Requirements • Low area • Low power consumption • High timing performance TDC 50 µm Nicolas. Roy 6@USherbrooke. ca m µ 0 5 6
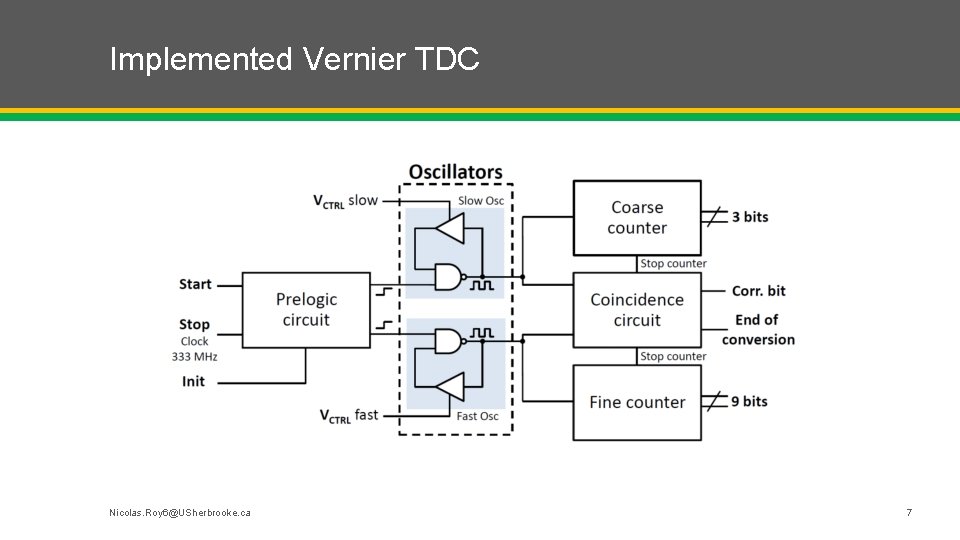
Implemented Vernier TDC Nicolas. Roy 6@USherbrooke. ca 7
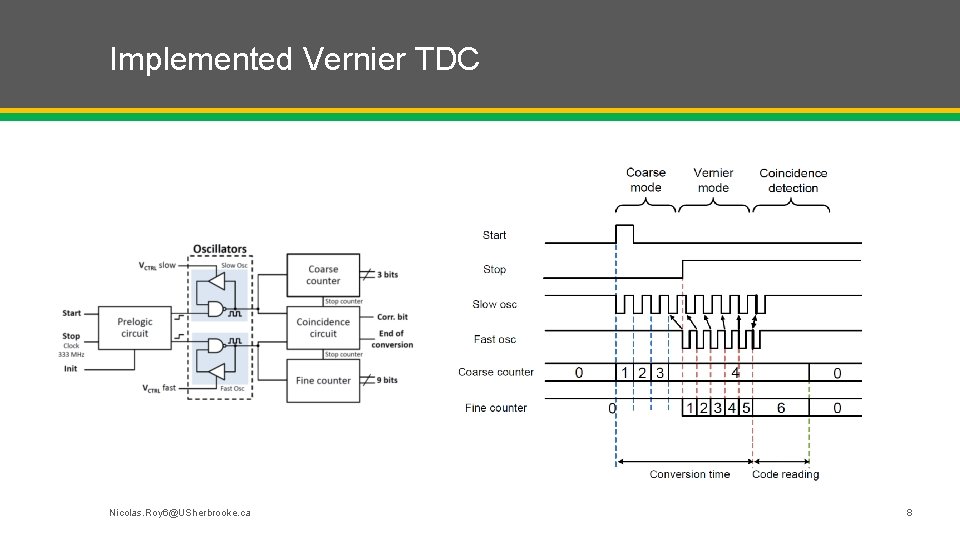
Implemented Vernier TDC Nicolas. Roy 6@USherbrooke. ca 8
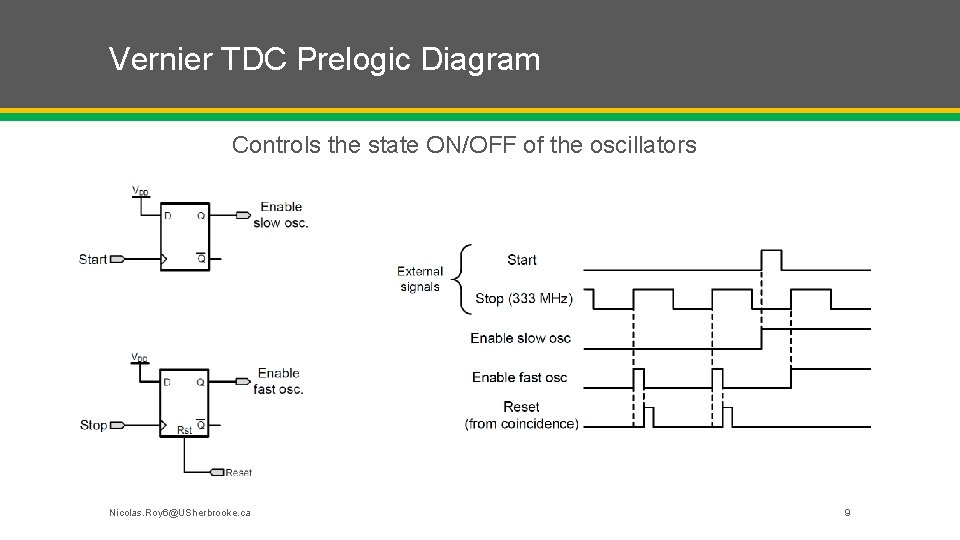
Vernier TDC Prelogic Diagram Controls the state ON/OFF of the oscillators Nicolas. Roy 6@USherbrooke. ca 9
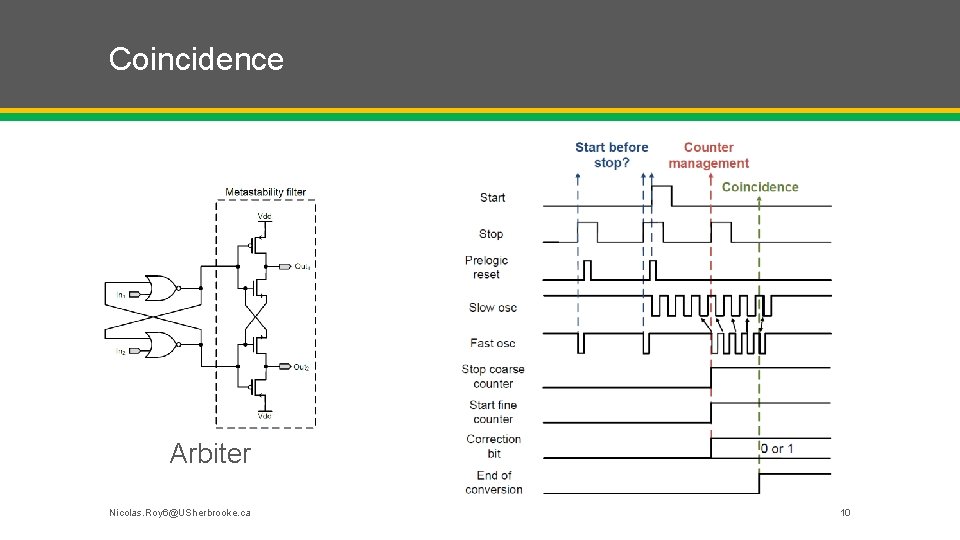
Coincidence Arbiter Nicolas. Roy 6@USherbrooke. ca 10
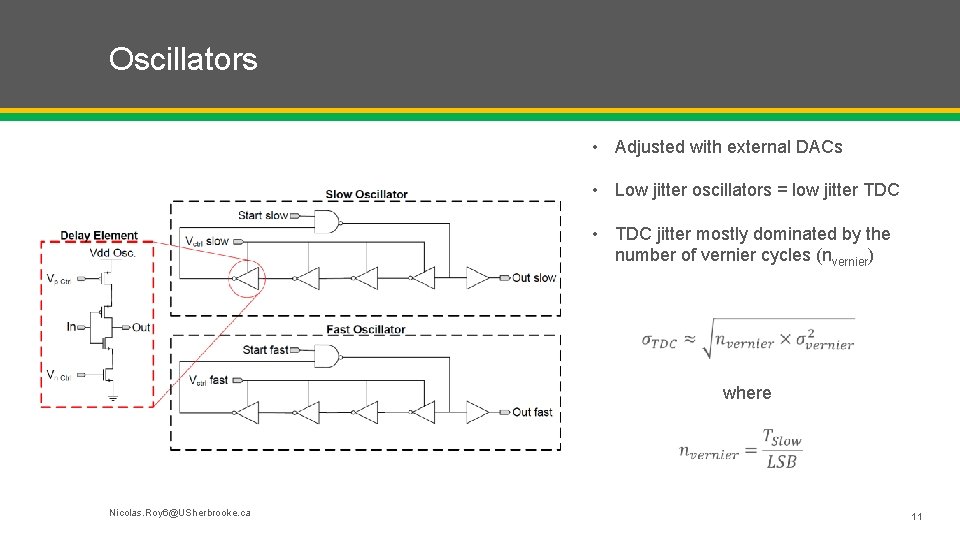
Oscillators • Adjusted with external DACs • Low jitter oscillators = low jitter TDC • TDC jitter mostly dominated by the number of vernier cycles (nvernier) where Nicolas. Roy 6@USherbrooke. ca 11
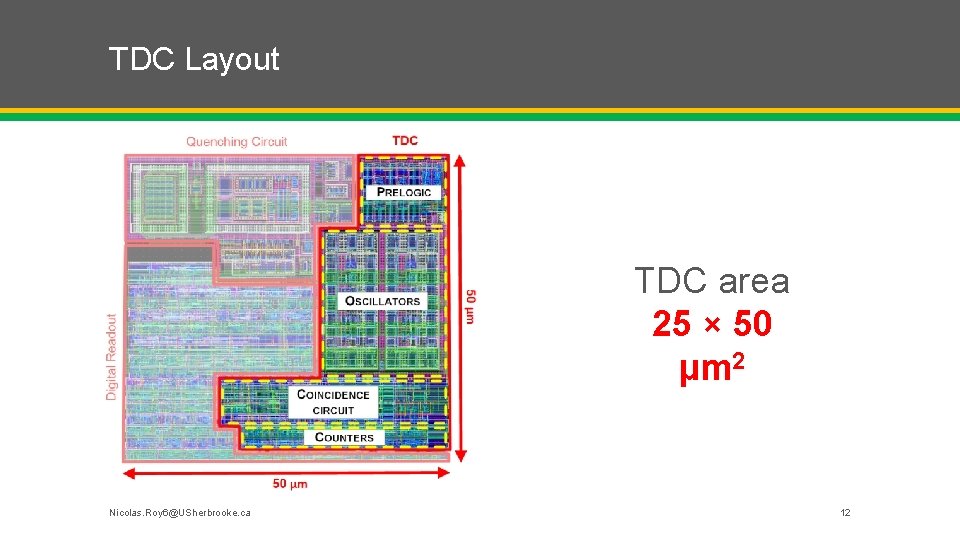
TDC Layout TDC area 25 × 50 µm 2 Nicolas. Roy 6@USherbrooke. ca 12
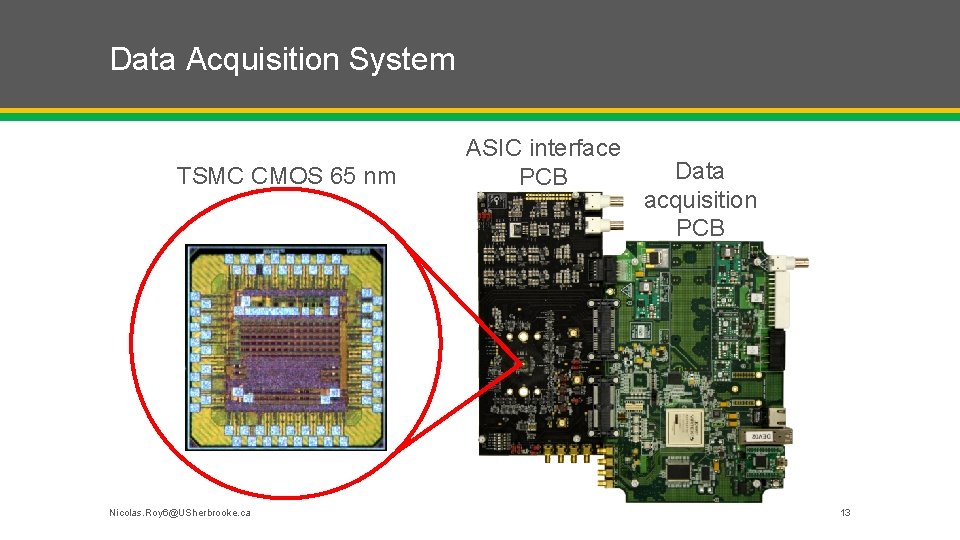
Data Acquisition System TSMC CMOS 65 nm Nicolas. Roy 6@USherbrooke. ca ASIC interface PCB Data acquisition PCB 13
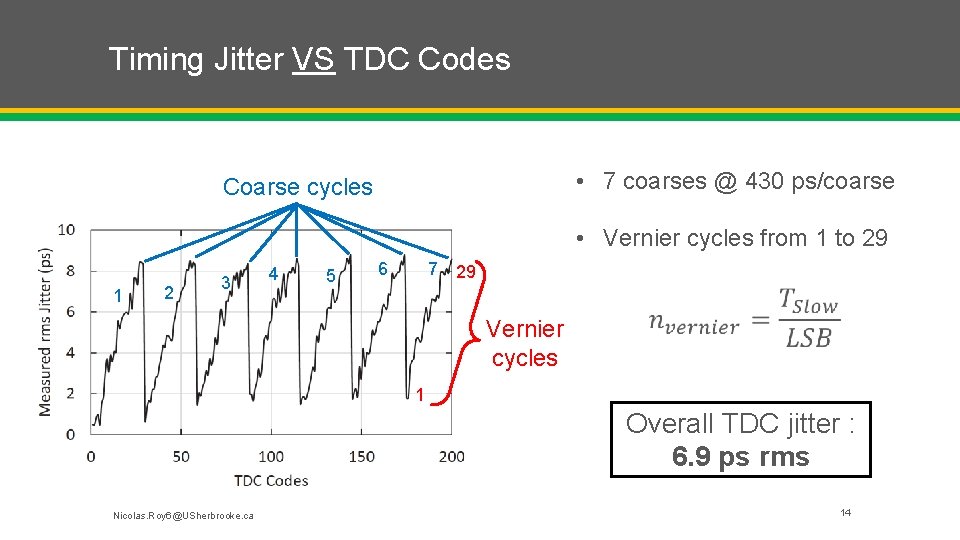
Timing Jitter VS TDC Codes • 7 coarses @ 430 ps/coarse Coarse cycles • Vernier cycles from 1 to 29 1 2 3 4 5 6 7 29 Vernier cycles 1 Overall TDC jitter : 6. 9 ps rms Nicolas. Roy 6@USherbrooke. ca 14
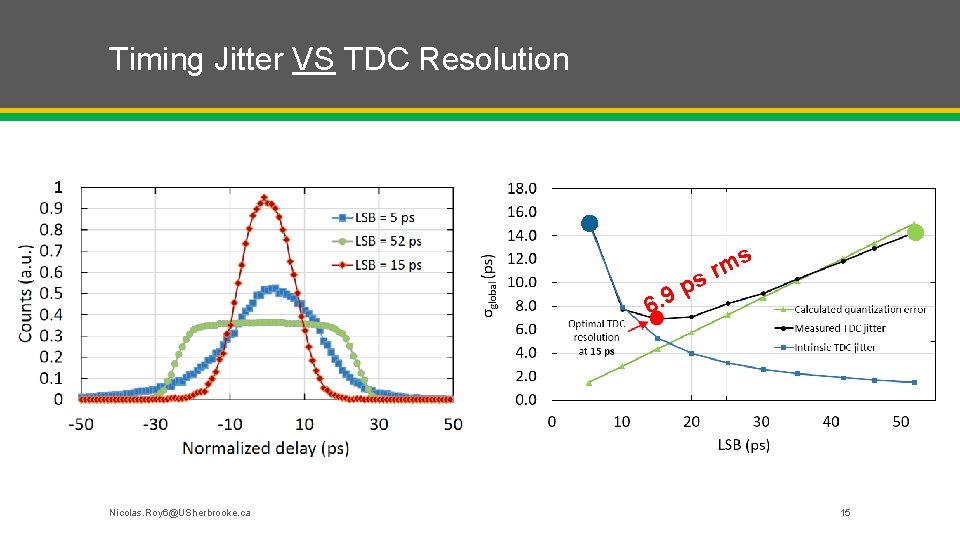
Timing Jitter VS TDC Resolution s m r s p 9 6. Nicolas. Roy 6@USherbrooke. ca 15
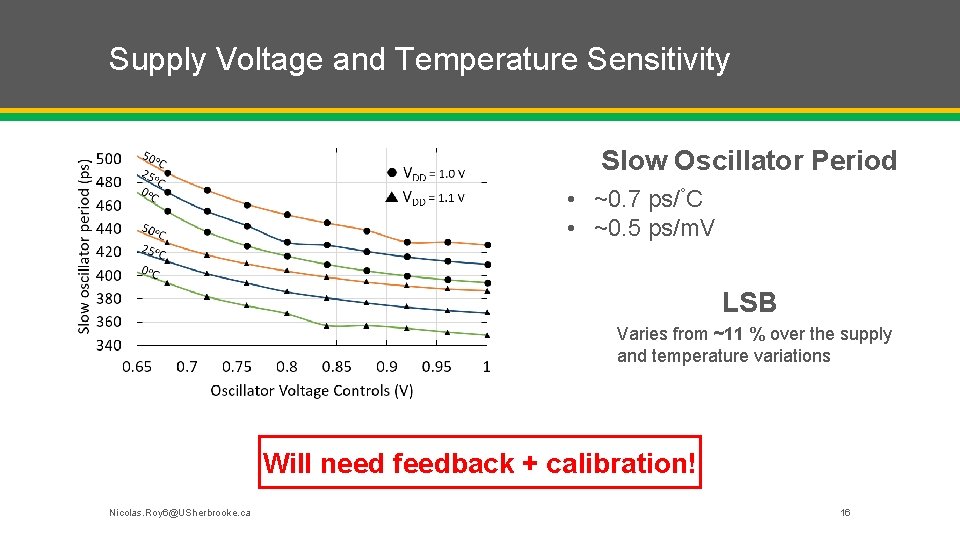
Supply Voltage and Temperature Sensitivity Slow Oscillator Period • ~0. 7 ps/°C • ~0. 5 ps/m. V LSB Varies from ~11 % over the supply and temperature variations Will need feedback + calibration! Nicolas. Roy 6@USherbrooke. ca 16
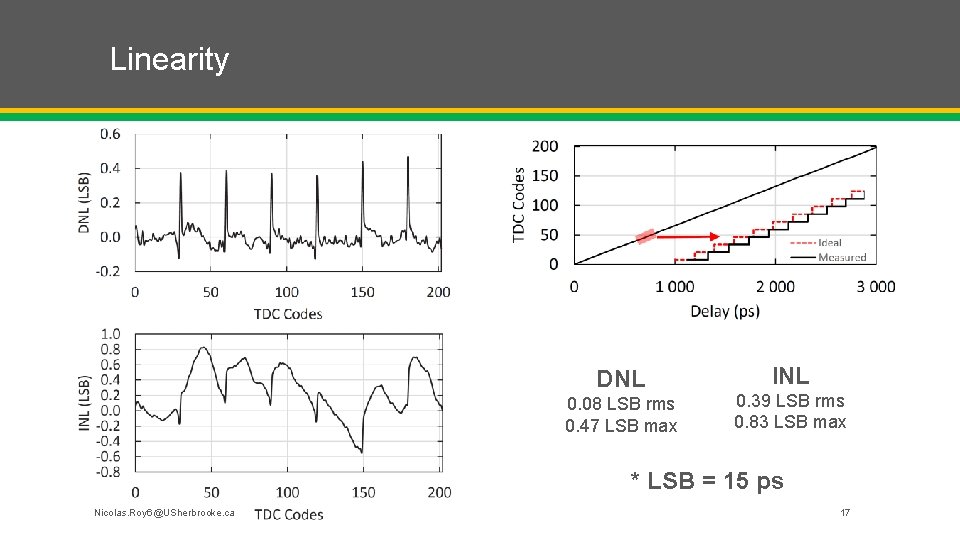
Linearity DNL 0. 08 LSB rms 0. 47 LSB max INL 0. 39 LSB rms 0. 83 LSB max * LSB = 15 ps Nicolas. Roy 6@USherbrooke. ca 17
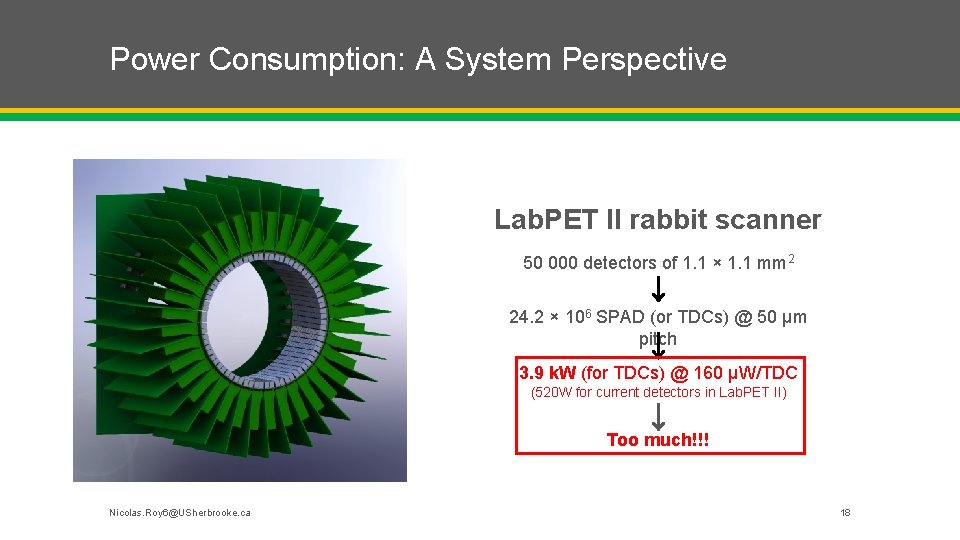
Power Consumption: A System Perspective Lab. PET II rabbit scanner 50 000 detectors of 1. 1 × 1. 1 mm 2 24. 2 × 106 SPAD (or TDCs) @ 50 µm pitch 3. 9 k. W (for TDCs) @ 160 µW/TDC (520 W for current detectors in Lab. PET II) Too much!!! Nicolas. Roy 6@USherbrooke. ca 18
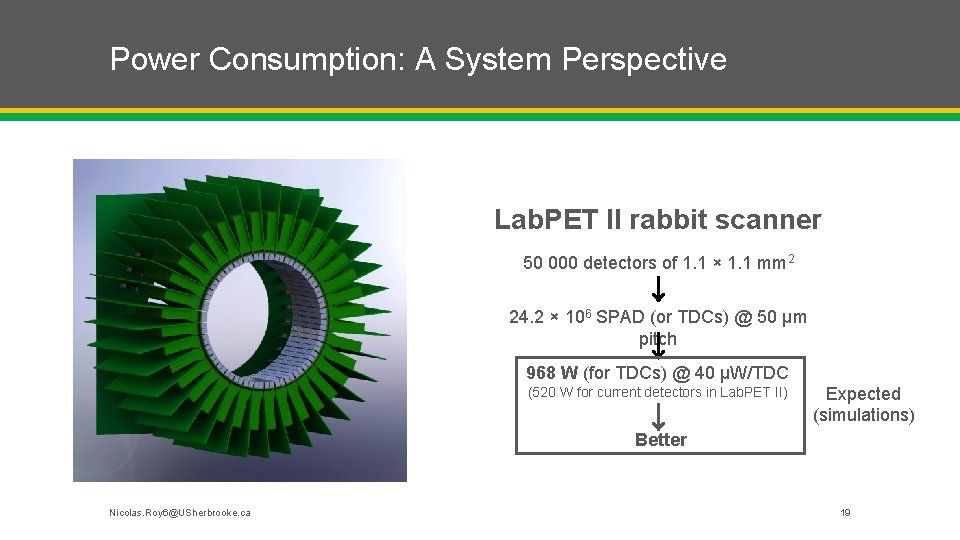
Power Consumption: A System Perspective Lab. PET II rabbit scanner 50 000 detectors of 1. 1 × 1. 1 mm 2 24. 2 × 106 SPAD (or TDCs) @ 50 µm pitch 968 W (for TDCs) @ 40 µW/TDC (520 W for current detectors in Lab. PET II) Expected (simulations) Better Nicolas. Roy 6@USherbrooke. ca 19
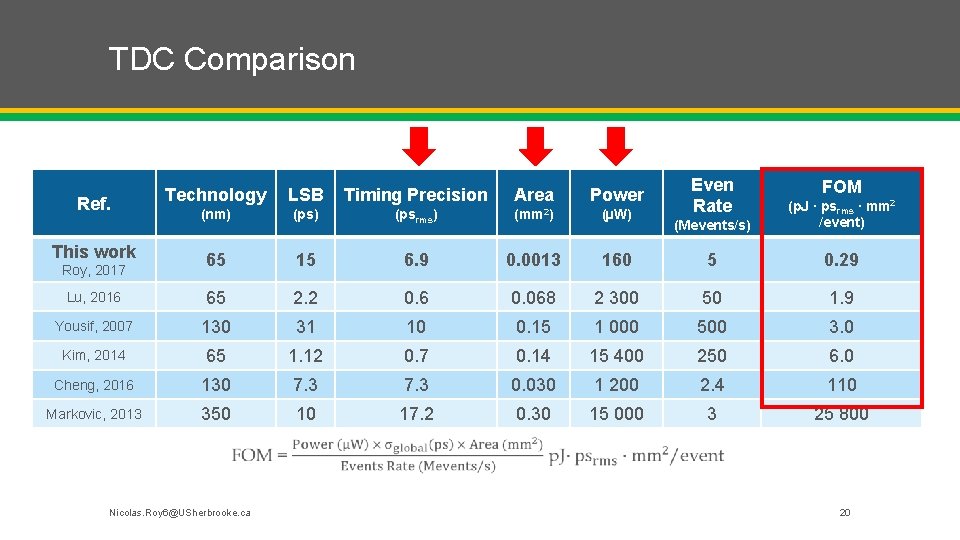
TDC Comparison Technology LSB Timing Precision Area Power (nm) (psrms) (mm 2) (µW) 65 15 6. 9 0. 0013 Lu, 2016 65 2. 2 0. 6 Yousif, 2007 130 31 Kim, 2014 65 Cheng, 2016 Markovic, 2013 Ref. This work Roy, 2017 Even Rate FOM (Mevents/s) (p. J ∙ psrms ∙ mm 2 /event) 160 5 0. 29 0. 068 2 300 50 1. 9 10 0. 15 1 000 500 3. 0 1. 12 0. 7 0. 14 15 400 250 6. 0 130 7. 3 0. 030 1 200 2. 4 110 350 10 17. 2 0. 30 15 000 3 25 800 Nicolas. Roy 6@USherbrooke. ca 20
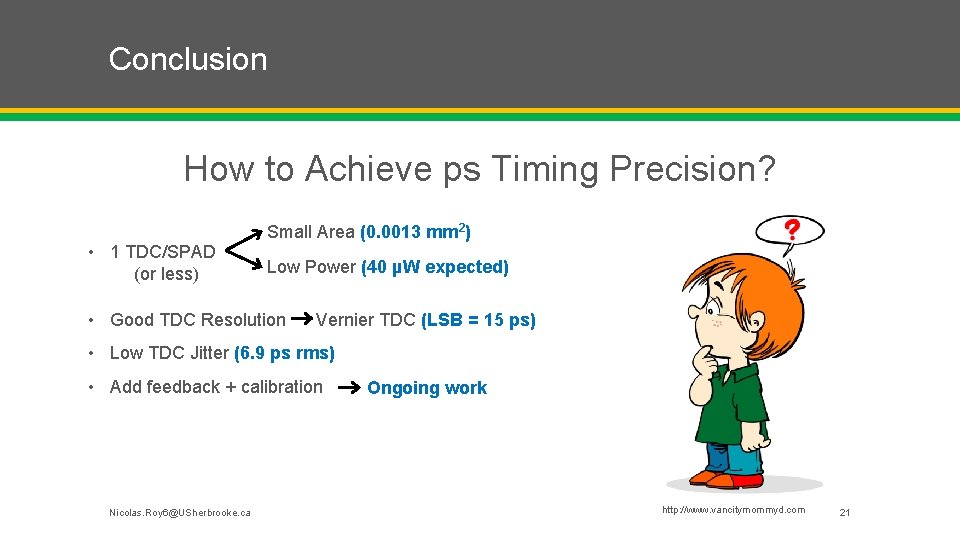
Conclusion How to Achieve ps Timing Precision? Small Area (0. 0013 mm 2) • 1 TDC/SPAD (or less) Low Power (40 µW expected) • Good TDC Resolution Vernier TDC (LSB = 15 ps) • Low TDC Jitter (6. 9 ps rms) • Add feedback + calibration Nicolas. Roy 6@USherbrooke. ca Ongoing work http: //www. vancitymommyd. com 21
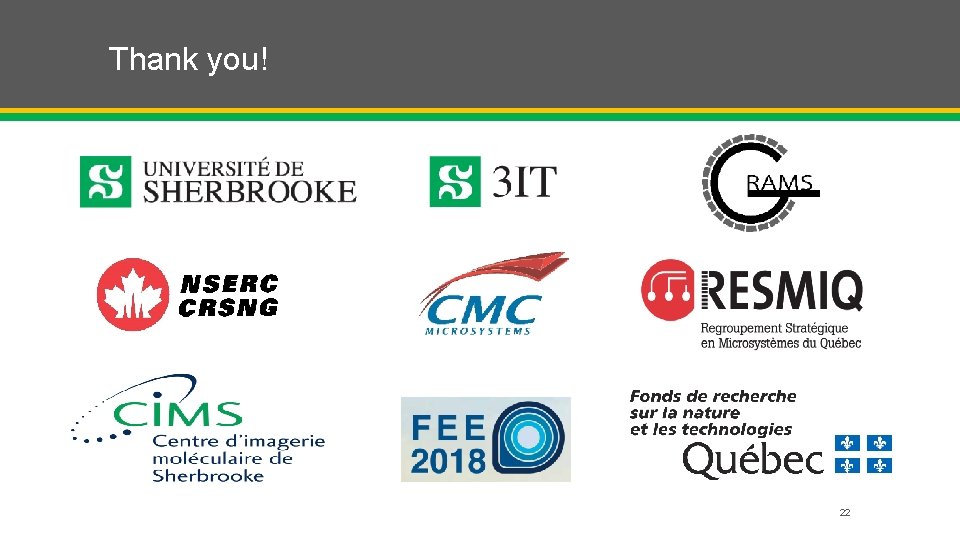
Thank you! 22
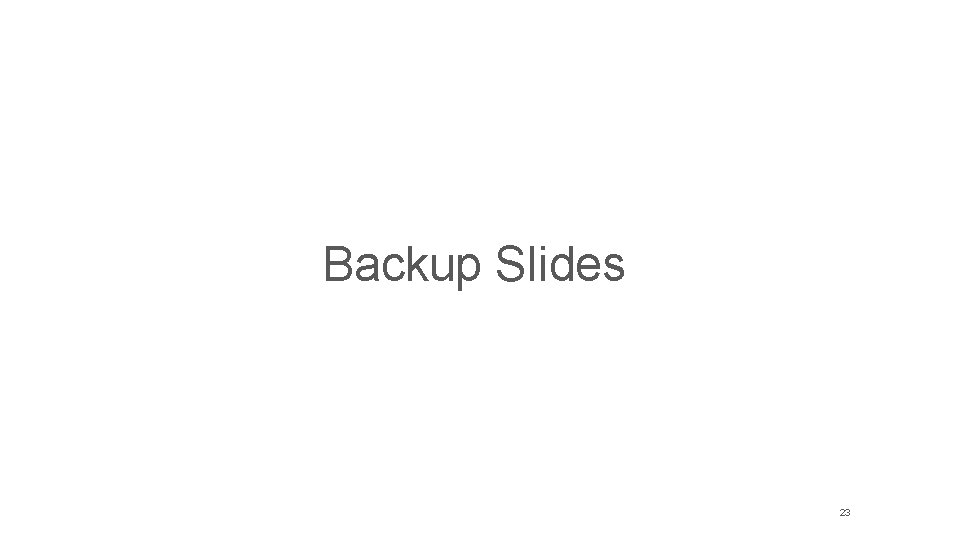
Backup Slides 23
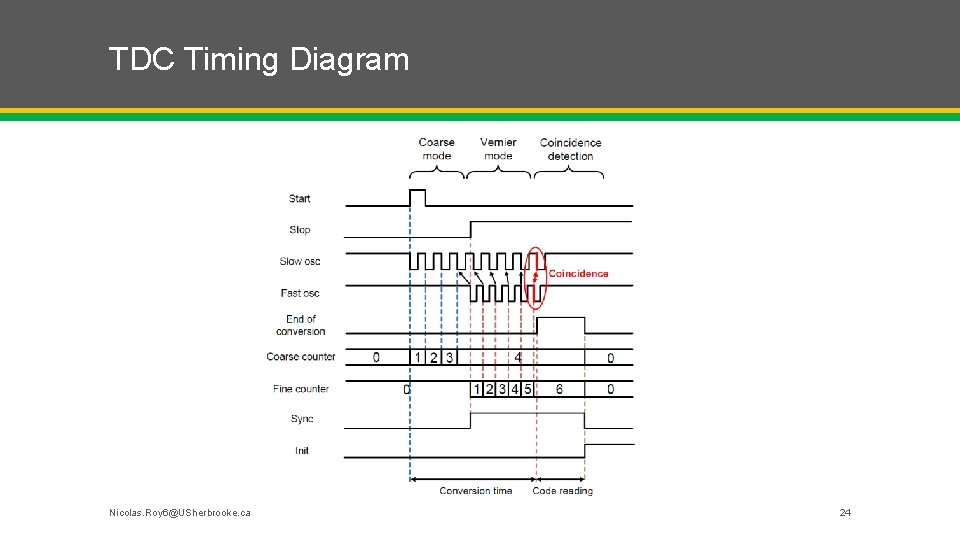
TDC Timing Diagram Nicolas. Roy 6@USherbrooke. ca 24
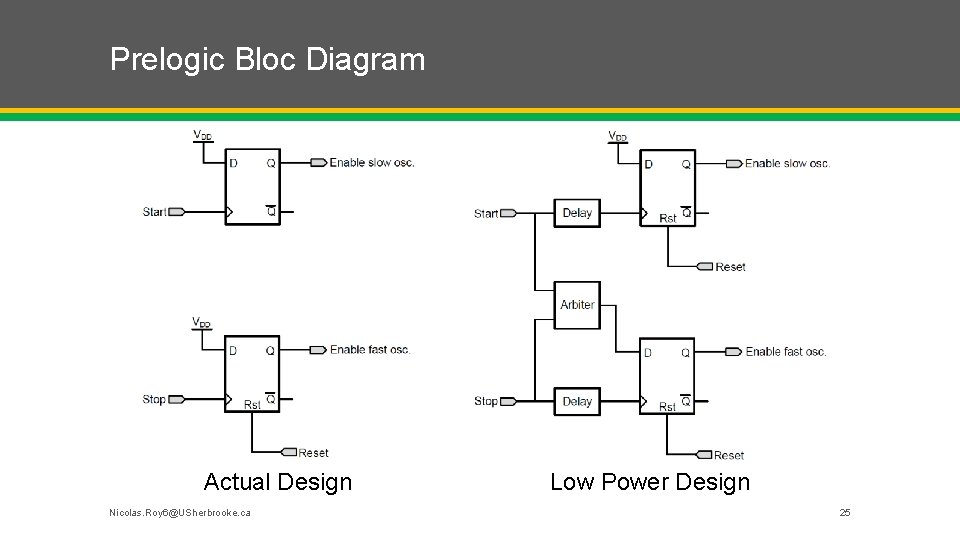
Prelogic Bloc Diagram Actual Design Nicolas. Roy 6@USherbrooke. ca Low Power Design 25
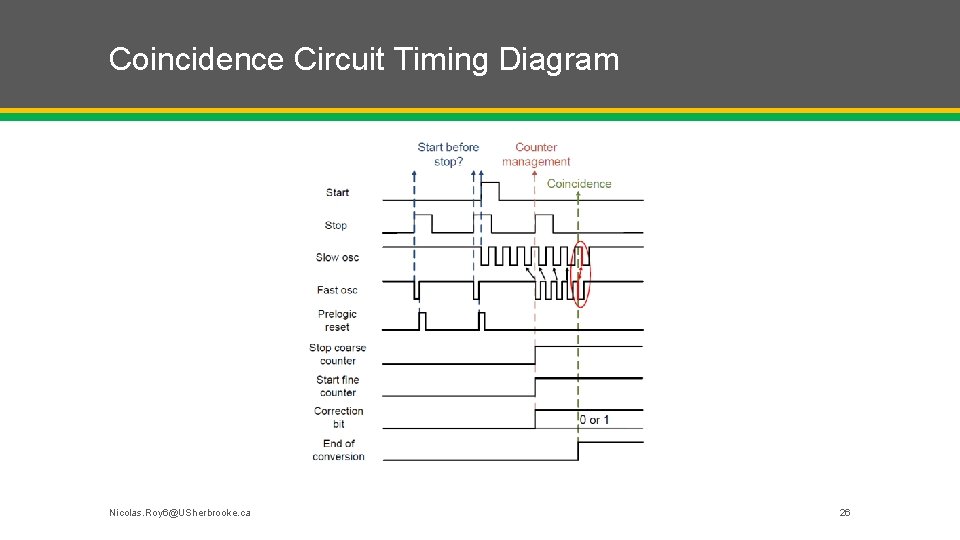
Coincidence Circuit Timing Diagram Nicolas. Roy 6@USherbrooke. ca 26
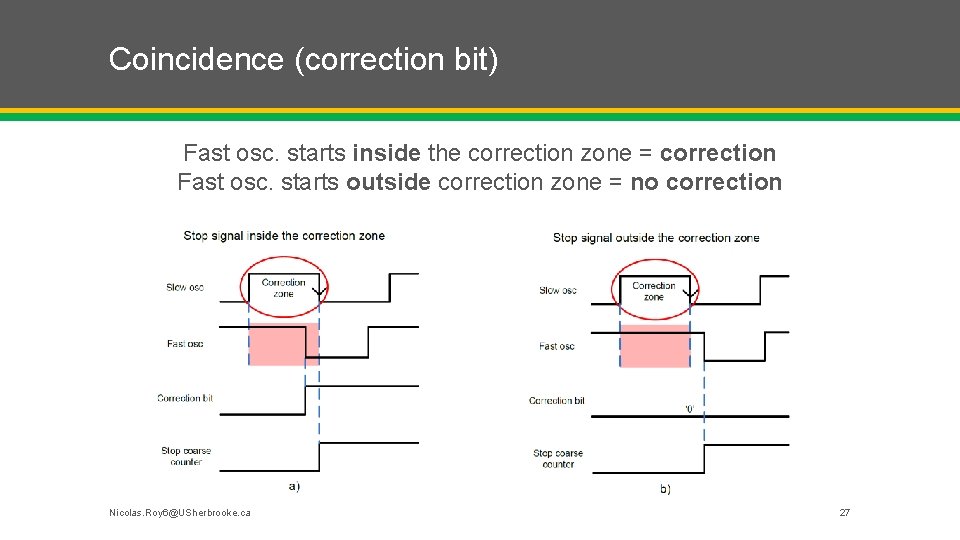
Coincidence (correction bit) Fast osc. starts inside the correction zone = correction Fast osc. starts outside correction zone = no correction Nicolas. Roy 6@USherbrooke. ca 27
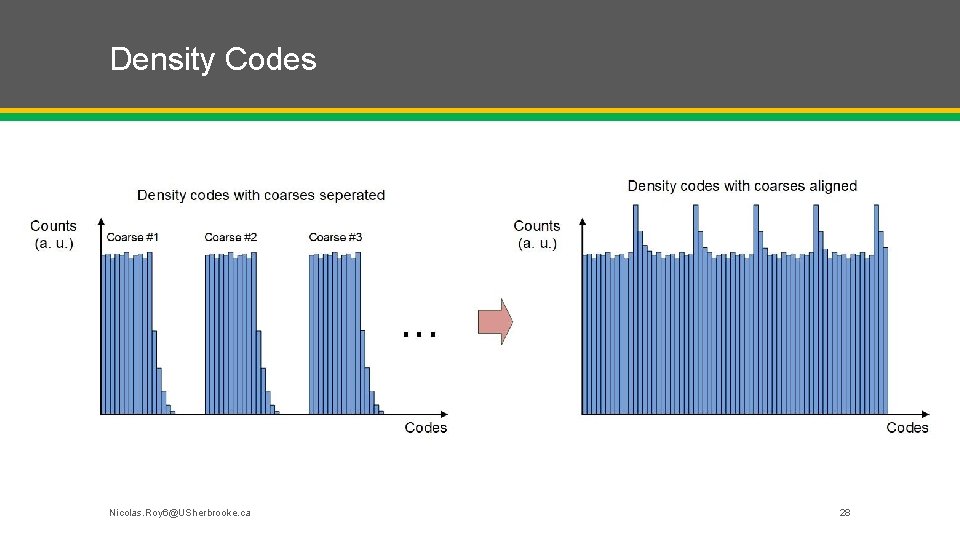
Density Codes Nicolas. Roy 6@USherbrooke. ca 28
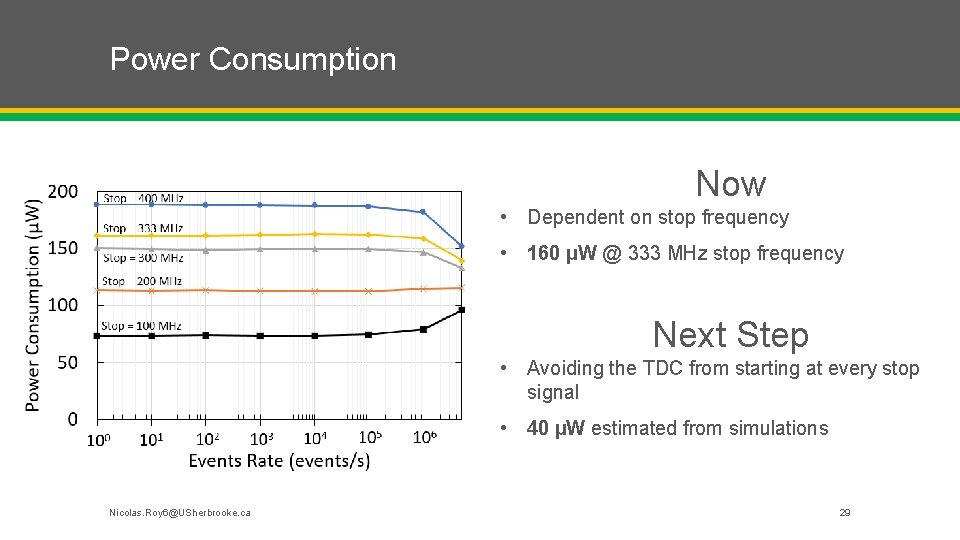
Power Consumption Now • Dependent on stop frequency • 160 µW @ 333 MHz stop frequency Next Step • Avoiding the TDC from starting at every stop signal • 40 µW estimated from simulations Nicolas. Roy 6@USherbrooke. ca 29
Buck converter and boost converter
Active power reactive power apparent power
Dominance continuum
Area of low pressure where air masses meet and rise
Mid = low + (high - low) / 2
High precision vs high accuracy
Low voltage hazards
Fspos
Novell typiska drag
Tack för att ni lyssnade bild
Ekologiskt fotavtryck
Varför kallas perioden 1918-1939 för mellankrigstiden?
En lathund för arbete med kontinuitetshantering
Adressändring ideell förening
Personlig tidbok fylla i
Sura för anatom
Förklara densitet för barn
Datorkunskap för nybörjare
Tack för att ni lyssnade bild
Mall för debattartikel
Delegerande ledarskap
Nyckelkompetenser för livslångt lärande
Påbyggnader för flakfordon
Kraft per area
Offentlig förvaltning
Urban torhamn
Presentera för publik crossboss
Argument för teckenspråk som minoritetsspråk
Kanaans land
Treserva lathund