Identification of reaction ratedetermining steps at SOFC electrodes
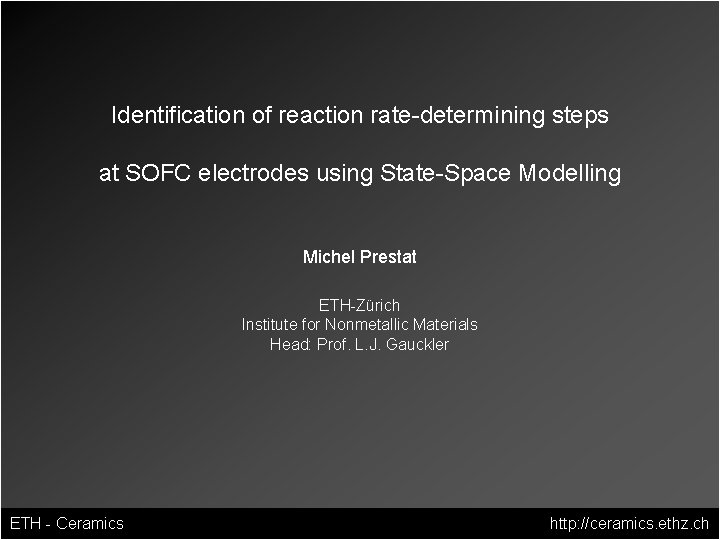
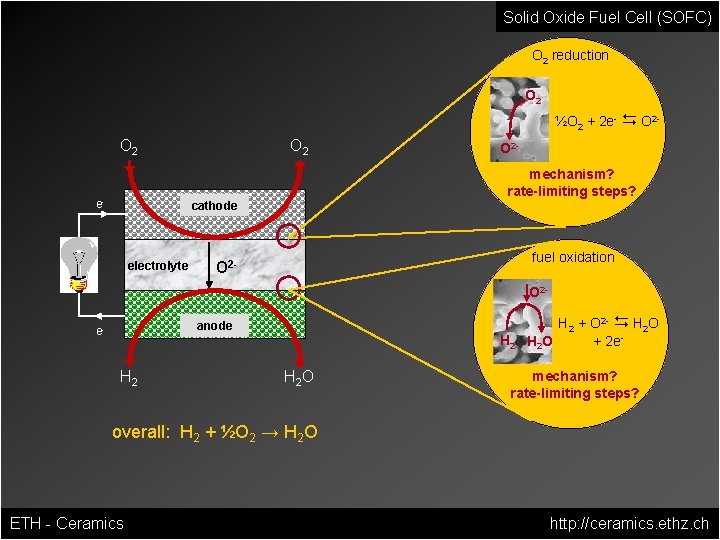
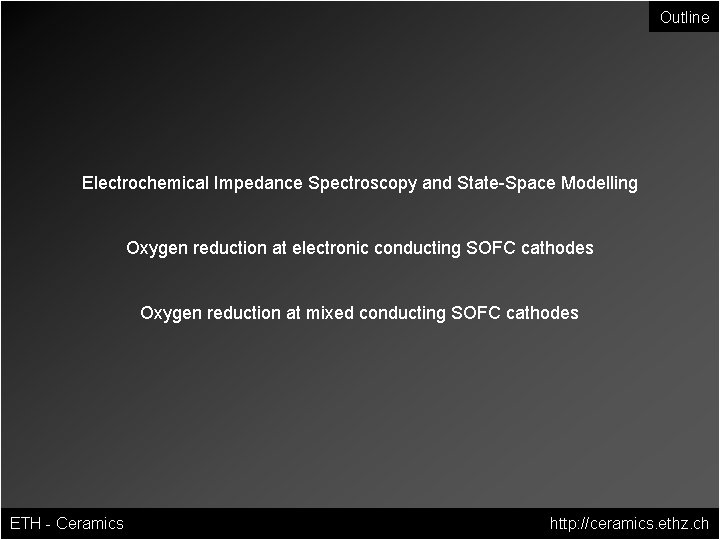
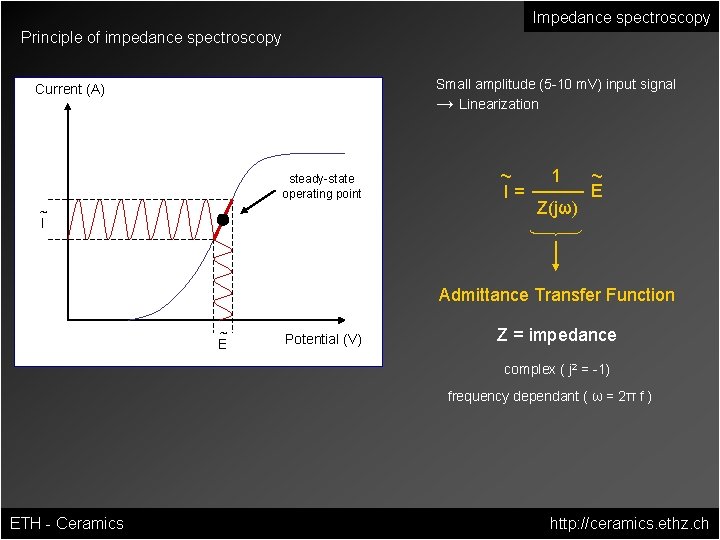
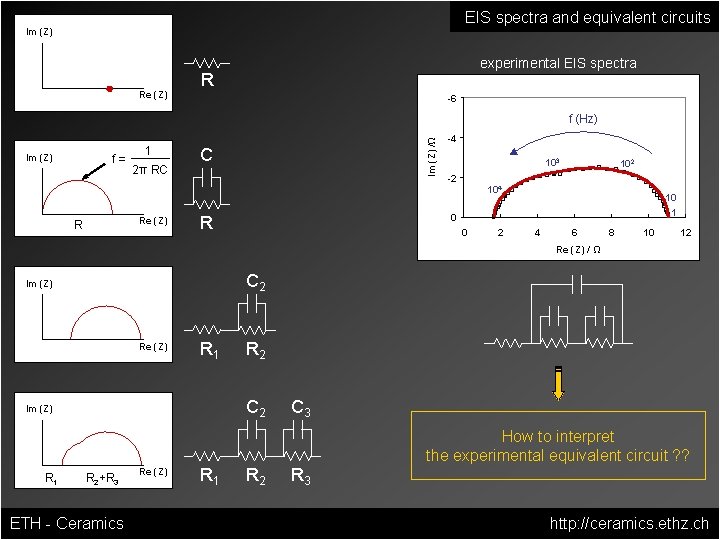
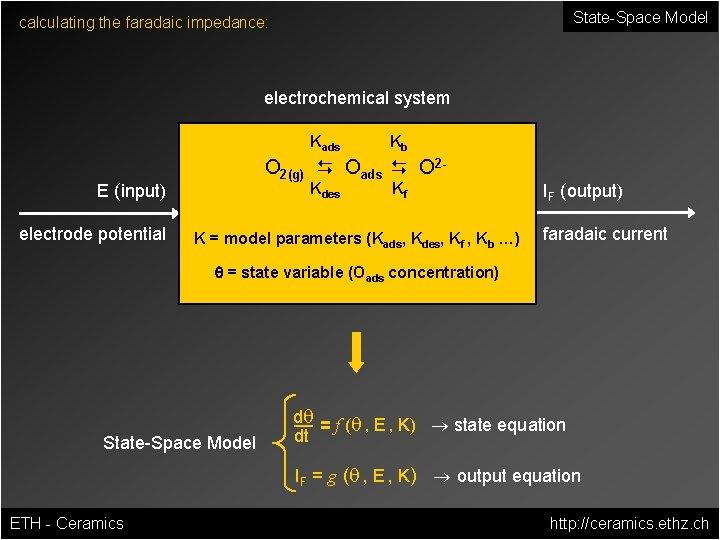
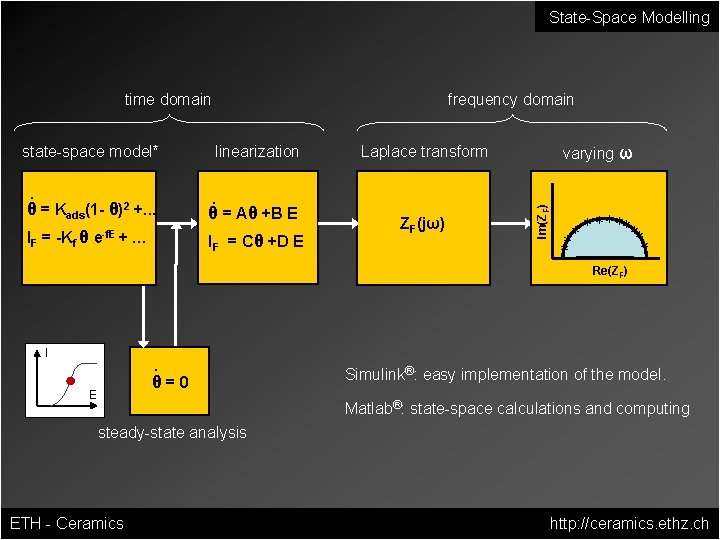
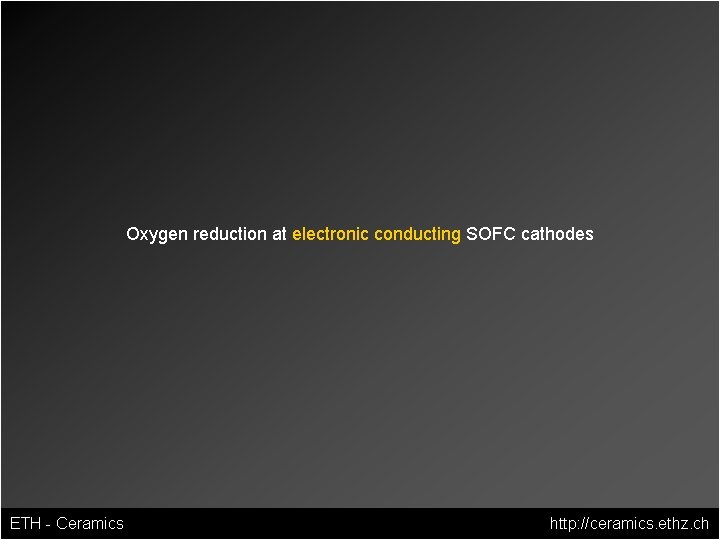
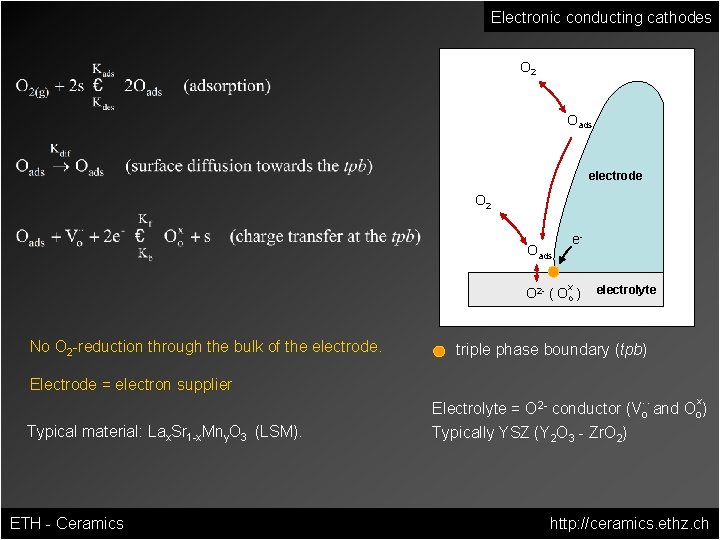
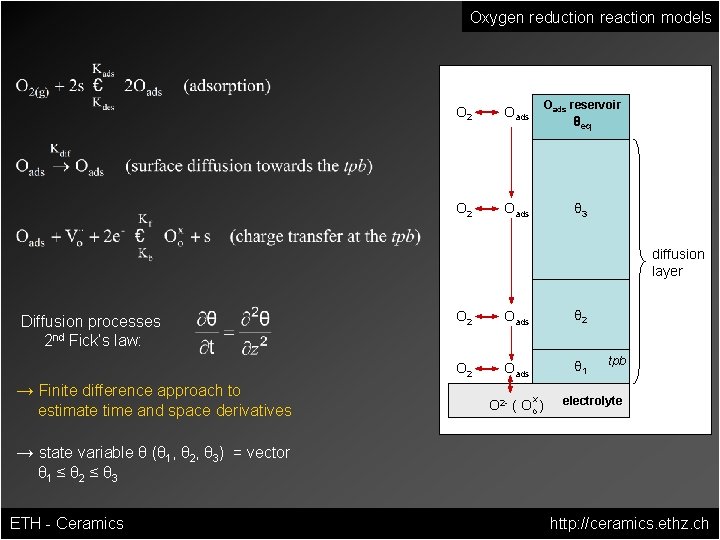
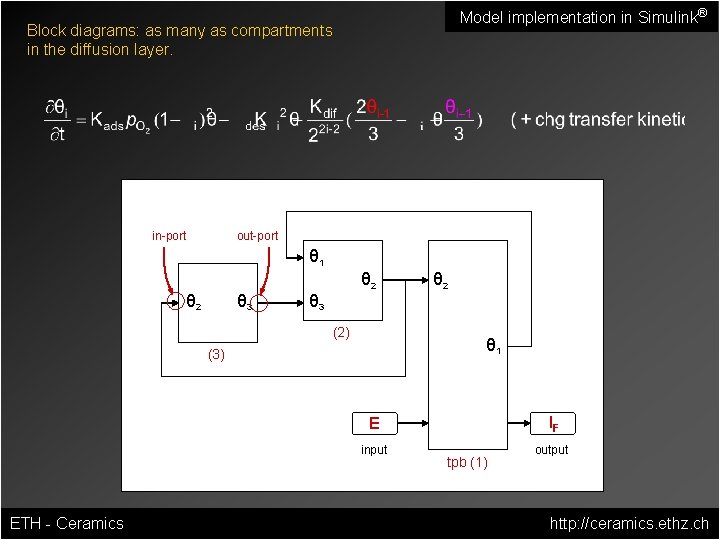
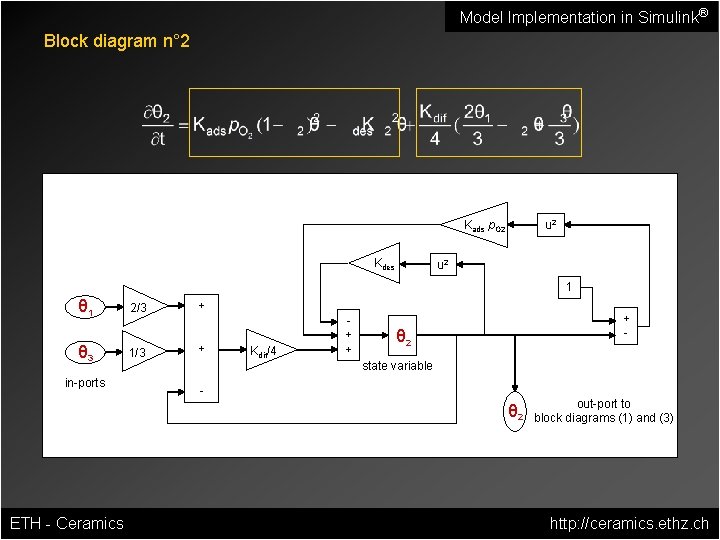
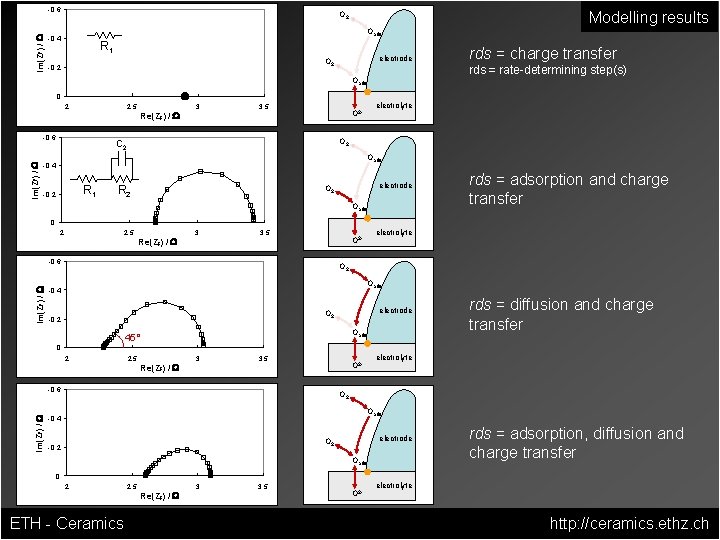
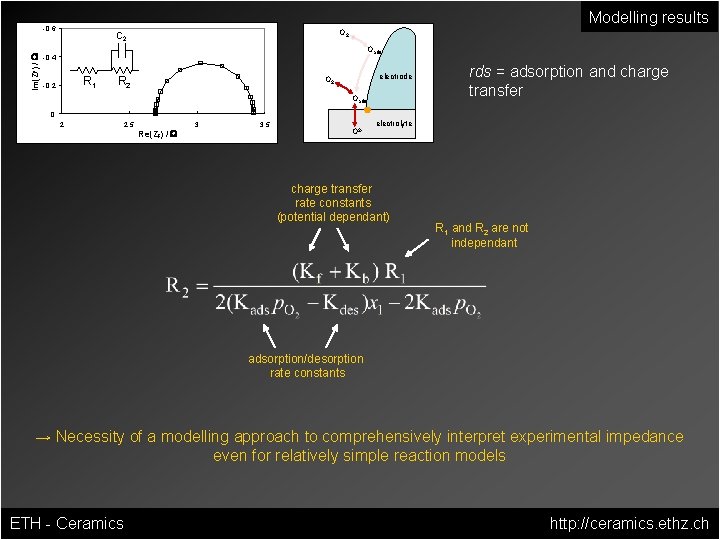
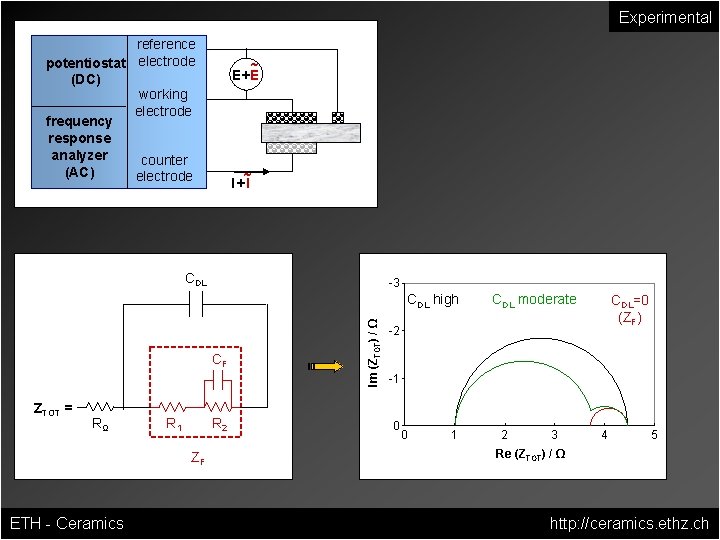
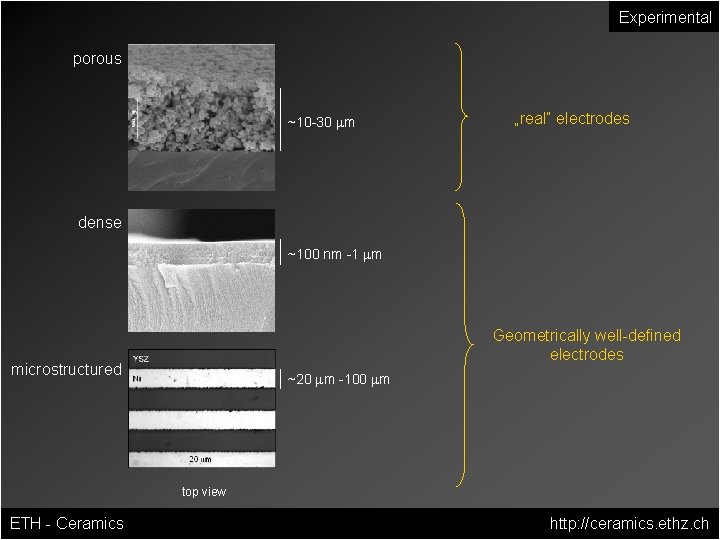
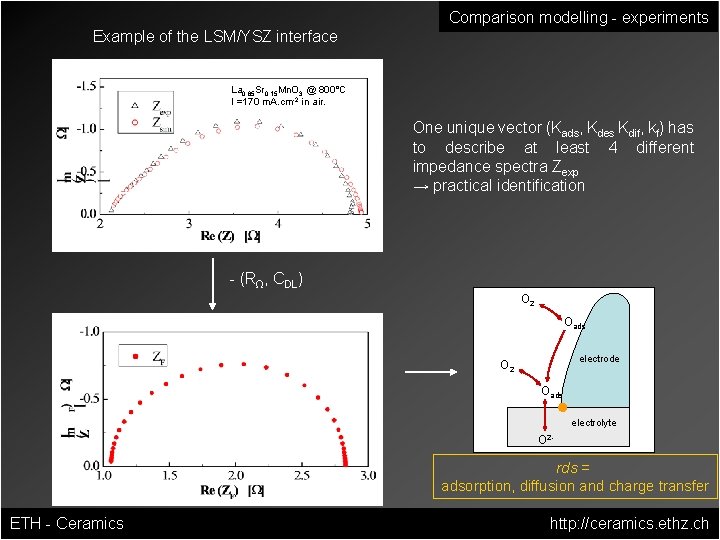
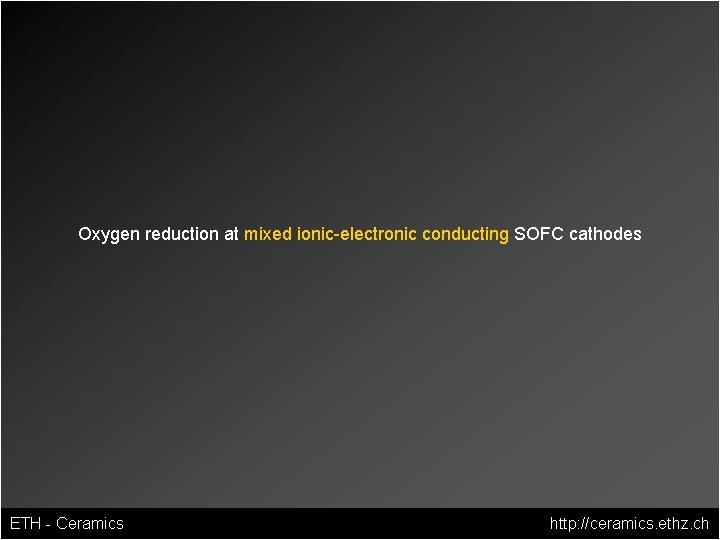
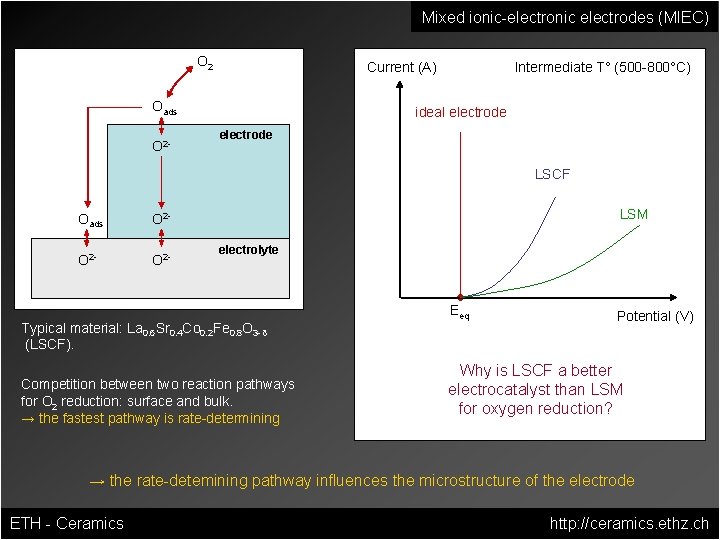
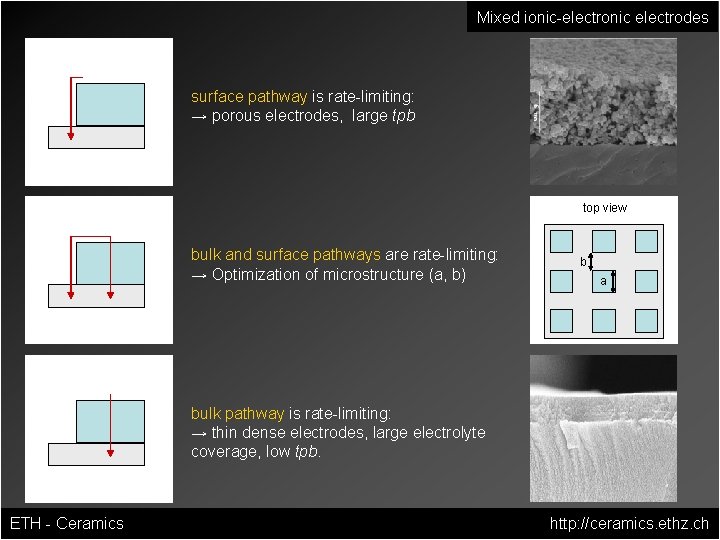
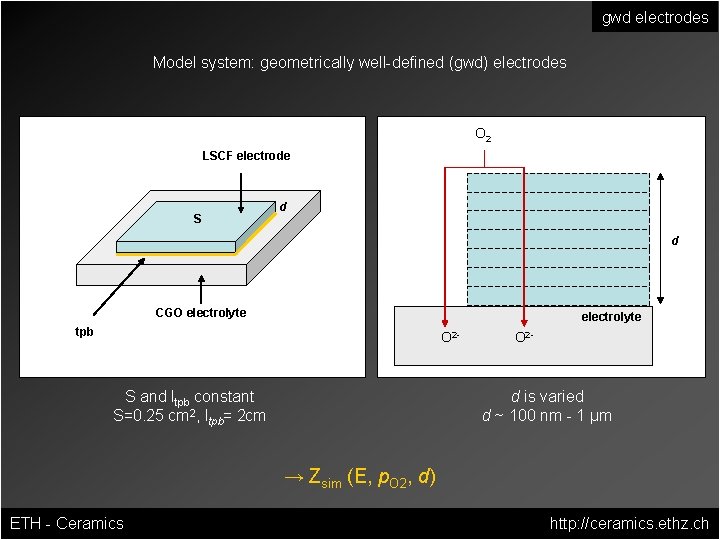
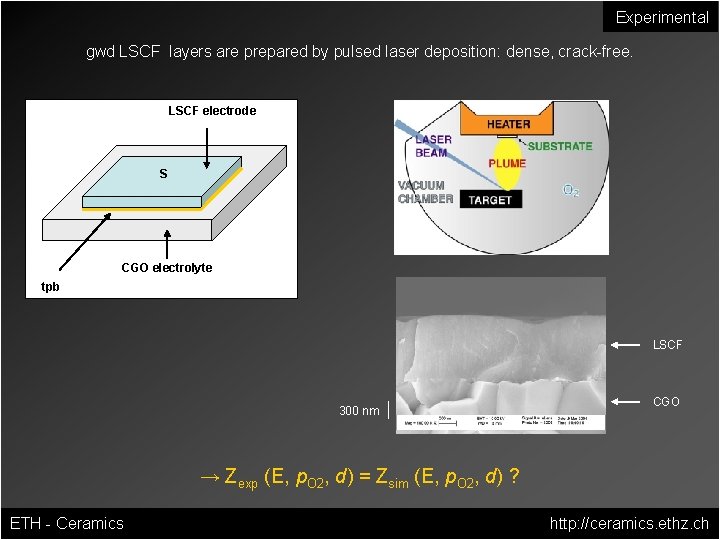
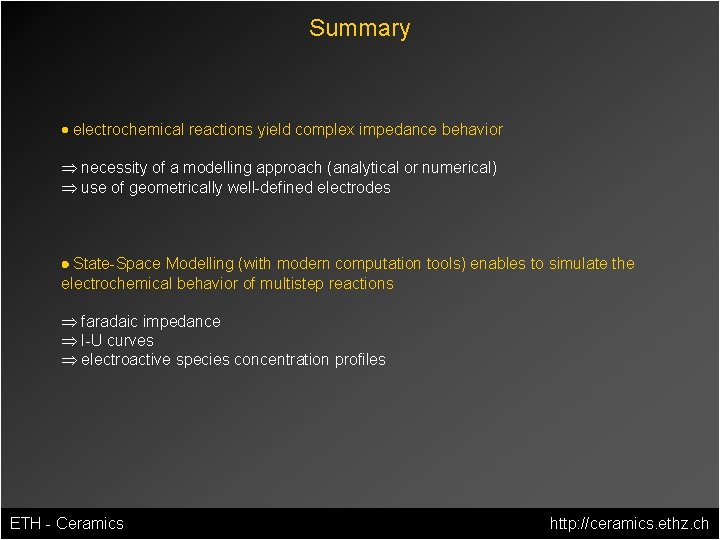
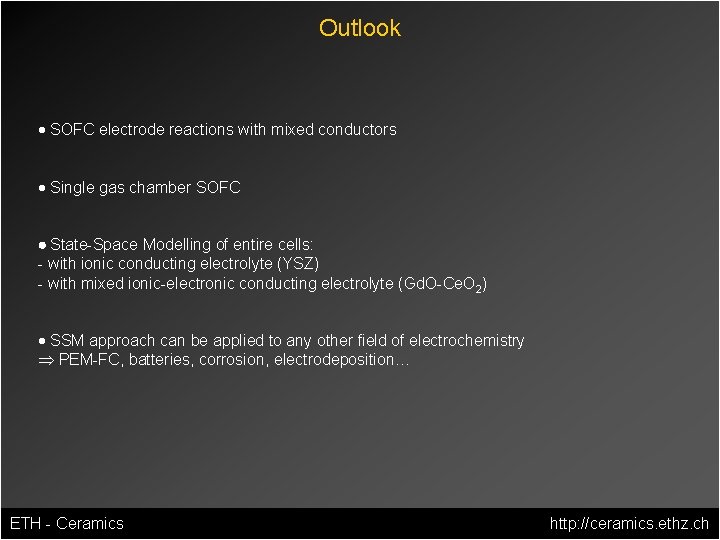
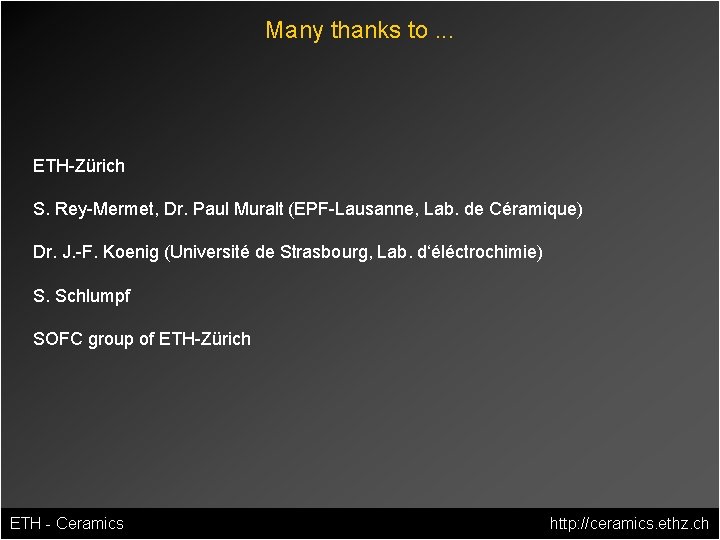
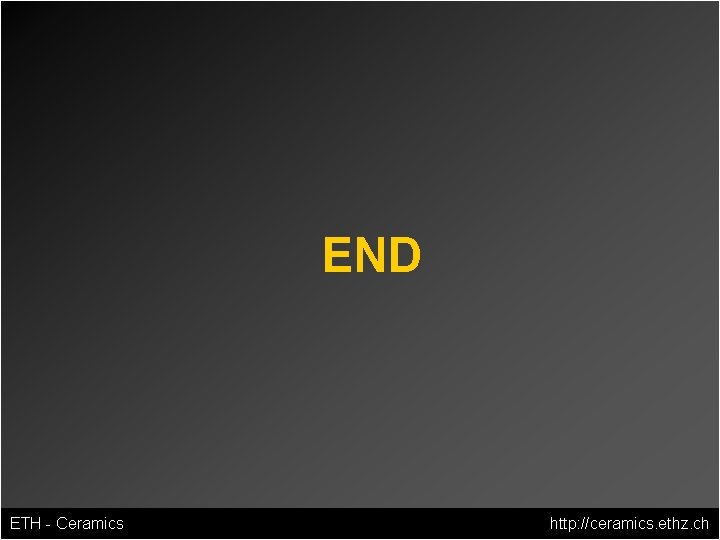
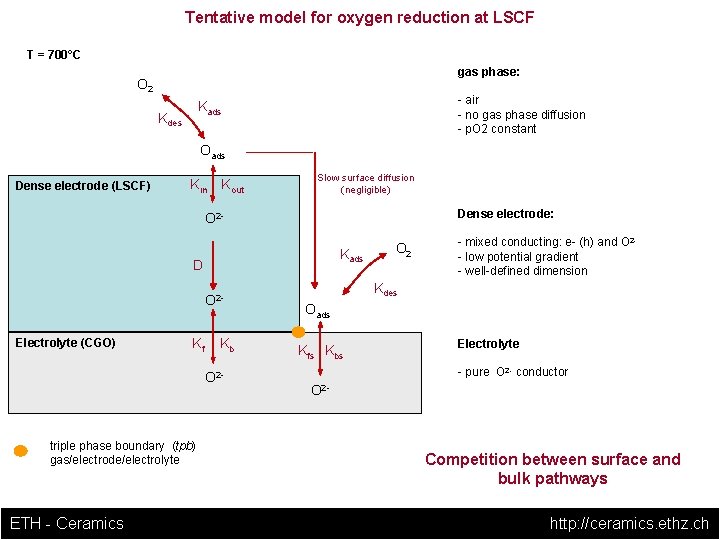
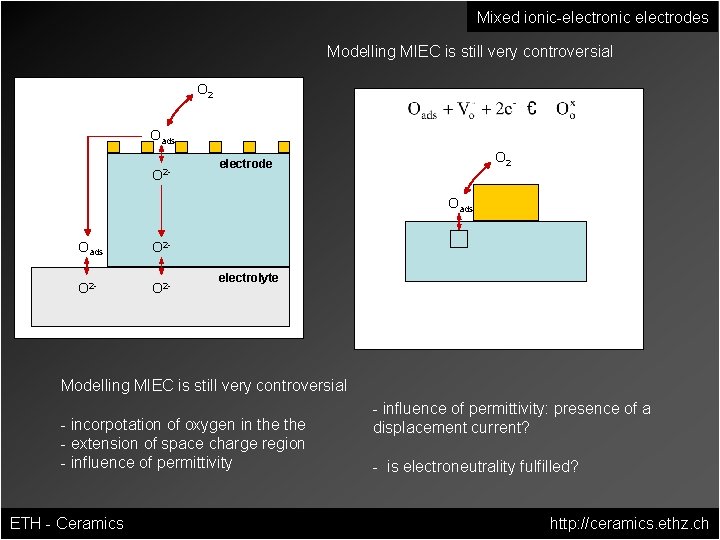
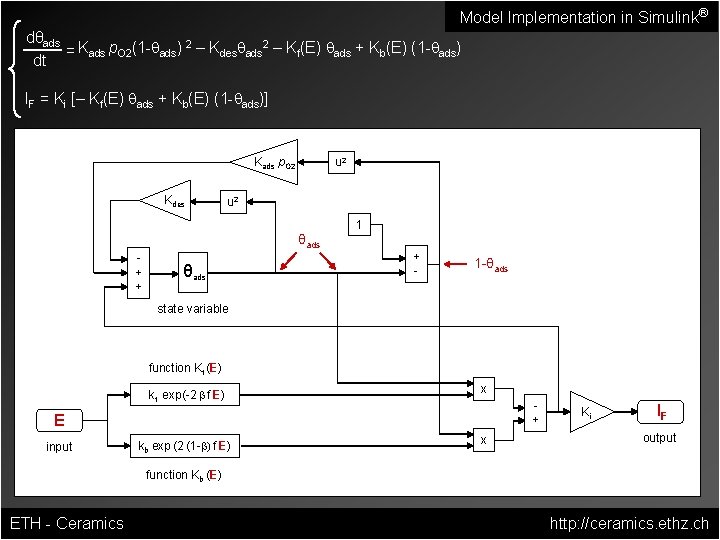
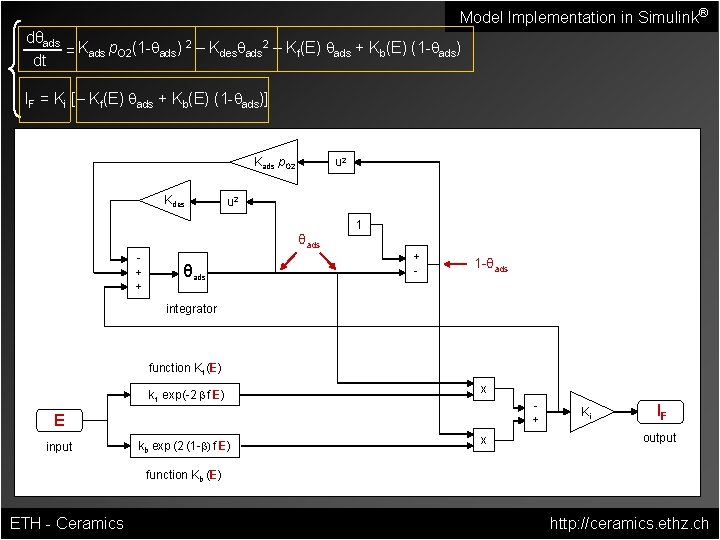
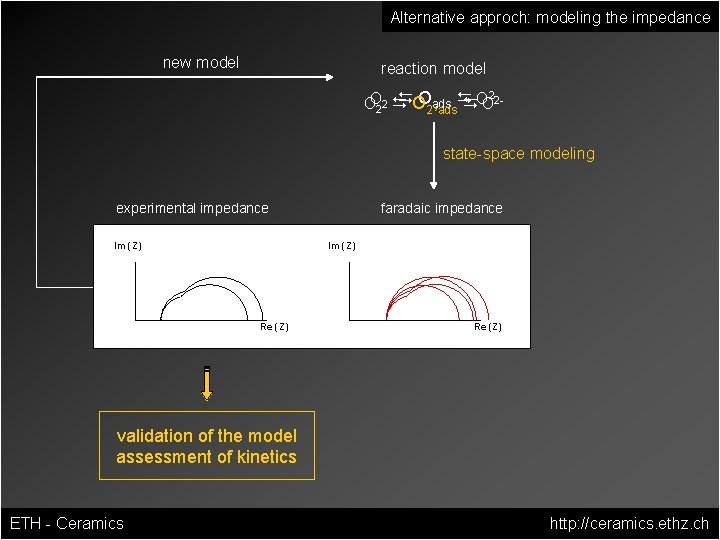
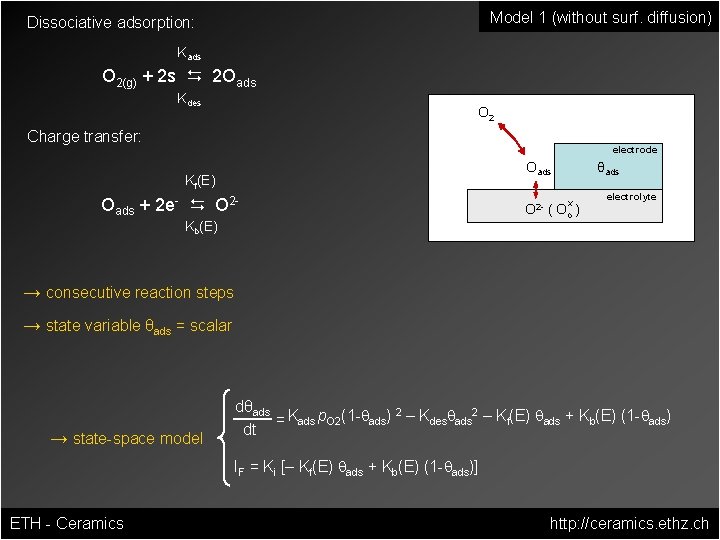
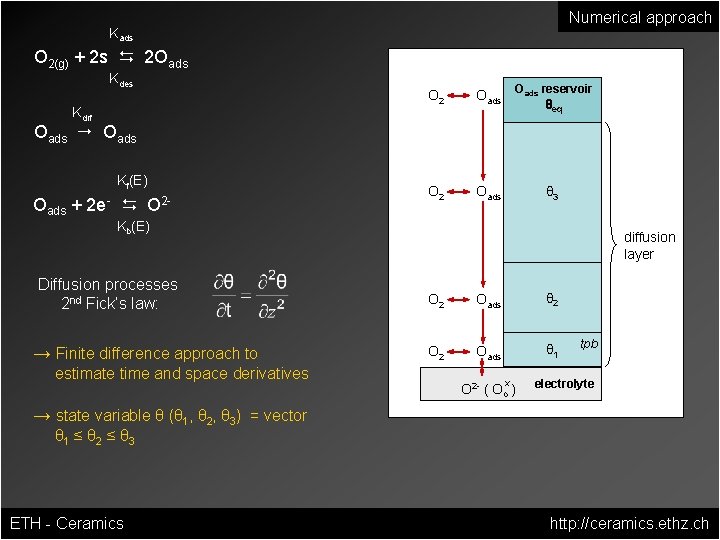
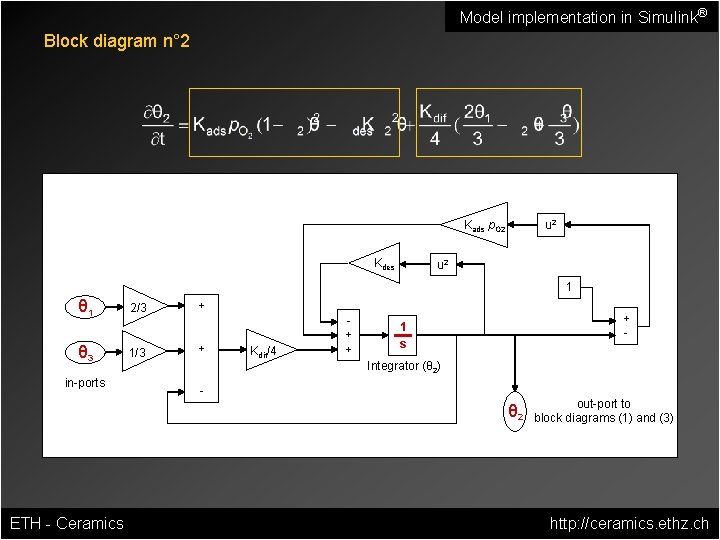
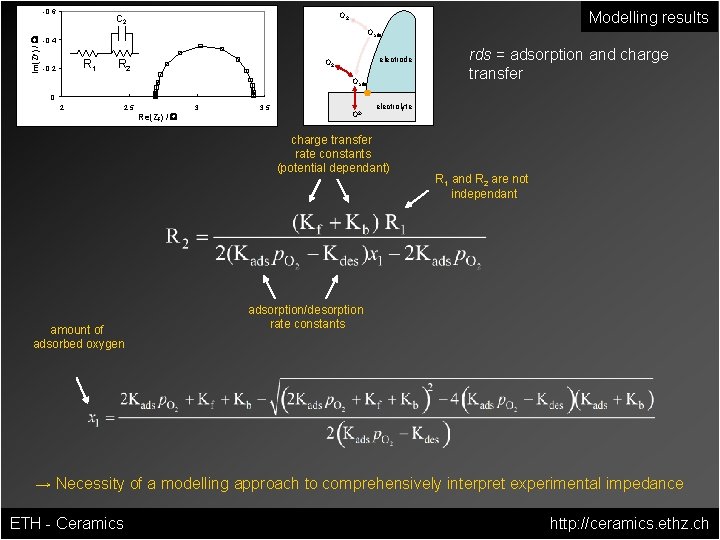
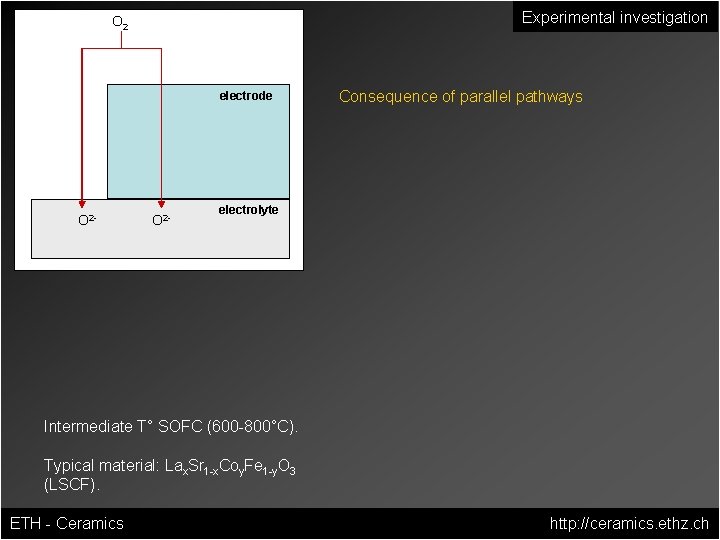
- Slides: 36
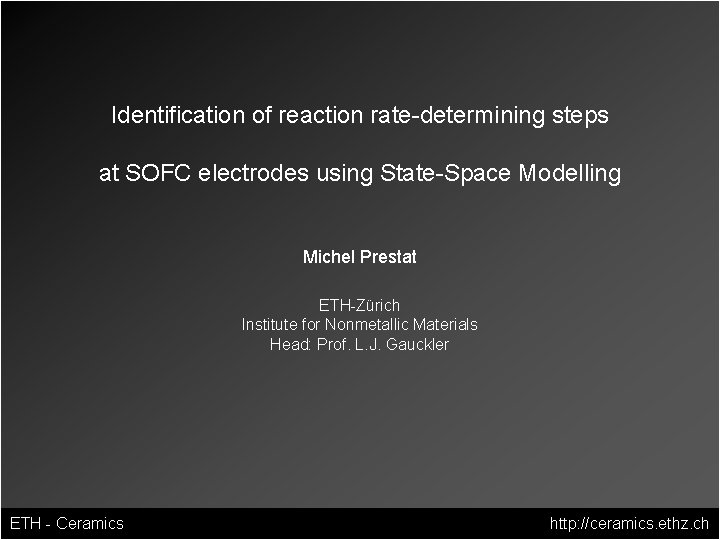
Identification of reaction rate-determining steps at SOFC electrodes using State-Space Modelling Michel Prestat ETH-Zürich Institute for Nonmetallic Materials Head: Prof. L. J. Gauckler ETH - Ceramics http: //ceramics. ethz. ch
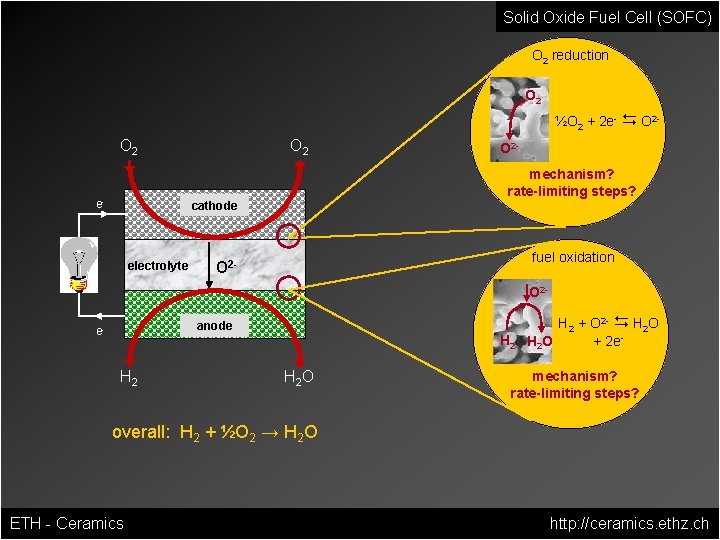
Solid Oxide Fuel Cell (SOFC) O 2 reduction O 2 ½O 2 + 2 e- D O 2 - O 2 e- O 2 mechanism? rate-limiting steps? cathode electrolyte O 2 - fuel oxidation O 2 - O 2 anode e- H 2 H 2 O H 2 + O 2 - D H 2 O + 2 e. H 2 O mechanism? rate-limiting steps? overall: H 2 + ½O 2 → H 2 O ETH - Ceramics http: //ceramics. ethz. ch
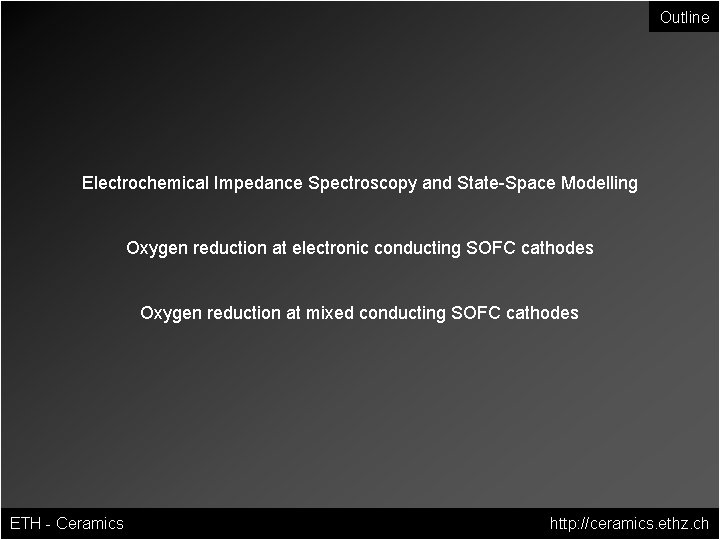
Outline Electrochemical Impedance Spectroscopy and State-Space Modelling Oxygen reduction at electronic conducting SOFC cathodes Oxygen reduction at mixed conducting SOFC cathodes ETH - Ceramics http: //ceramics. ethz. ch
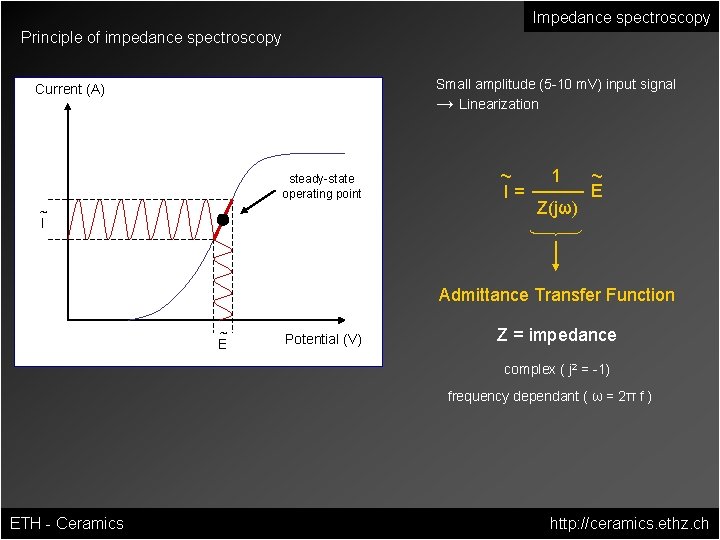
Impedance spectroscopy Principle of impedance spectroscopy Small amplitude (5 -10 m. V) input signal → Linearization Current (A) steady-state operating point ~ I= 1 Z(jω) ~ E Admittance Transfer Function ~ E Potential (V) Z = impedance complex ( j 2 = -1) frequency dependant ( ω = 2π f ) ETH - Ceramics http: //ceramics. ethz. ch
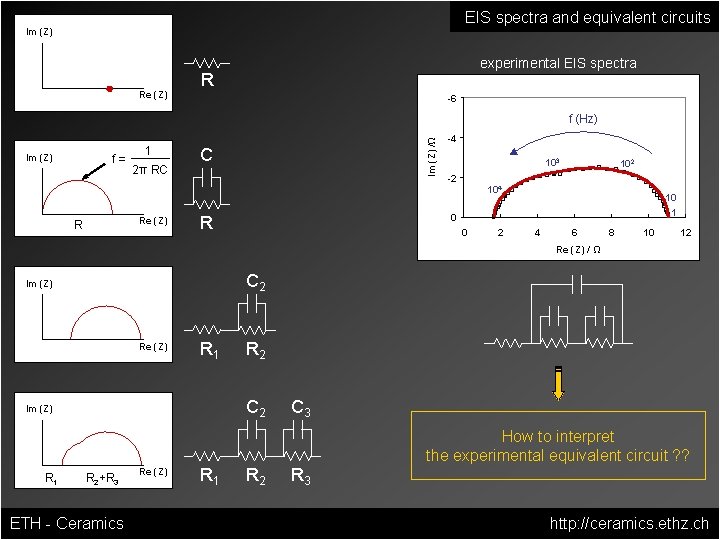
EIS spectra and equivalent circuits Im (Z) Re (Z) experimental EIS spectra R -6 f= Im (Z) 1 2π RC Im (Z) /Ω f (Hz) C -4 103 102 -2 104 Re (Z) R 10 1 0 R 0 2 4 6 8 10 12 Re (Z) / Ω C 2 Im (Z) Re (Z) R 1 C 2 Im (Z) R 1 R 2+R 3 ETH - Ceramics Re (Z) R 1 R 2 C 3 R 3 How to interpret the experimental equivalent circuit ? ? http: //ceramics. ethz. ch
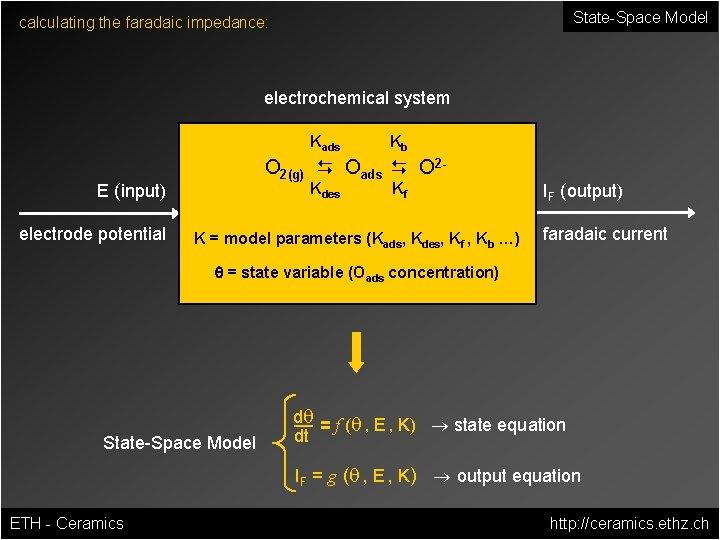
State-Space Model calculating the faradaic impedance: electrochemical system Kads O 2(g) D Oads D O 2 - E (input) electrode potential Kb Kdes Kf K = model parameters (Kads, Kdes, Kf , Kb …) IF (output) faradaic current q = state variable (Oads concentration) State-Space Model dq = f (q , E , K) ® state equation dt IF = g (q , E , K) ® output equation ETH - Ceramics http: //ceramics. ethz. ch
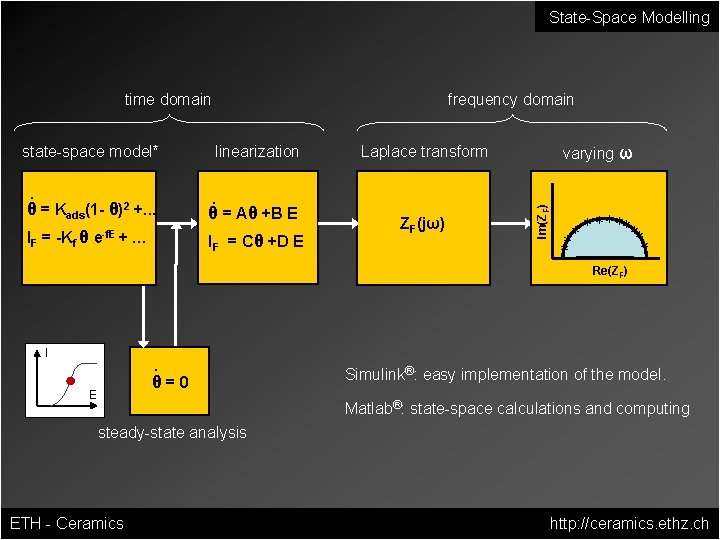
State-Space Modelling time domain . linearization Laplace transform . θ = Kads(1 - θ)2 +. . . θ = Aθ +B E IF = -Kf θ e-f. E + … IF = Cθ +D E ZF(jω) varying ω Im(ZF) state-space model* frequency domain * * ** ** * Re(ZF) I . θ=0 E Simulink®: easy implementation of the model. Matlab®: state-space calculations and computing steady-state analysis ETH - Ceramics http: //ceramics. ethz. ch
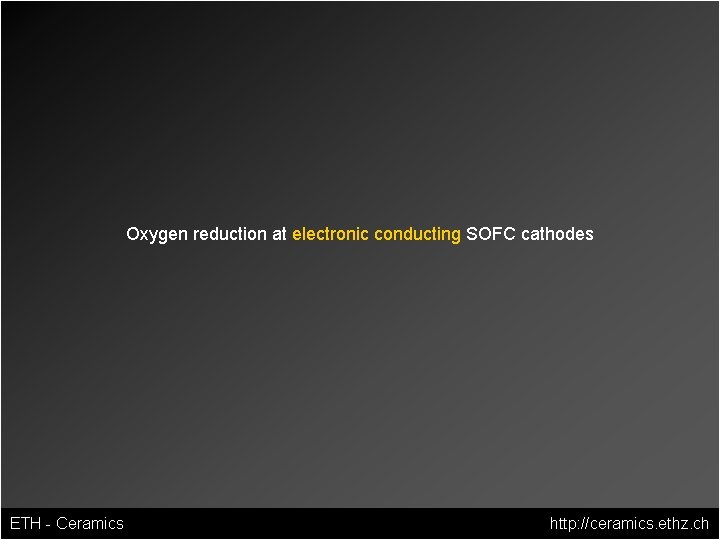
Oxygen reduction at electronic conducting SOFC cathodes ETH - Ceramics http: //ceramics. ethz. ch
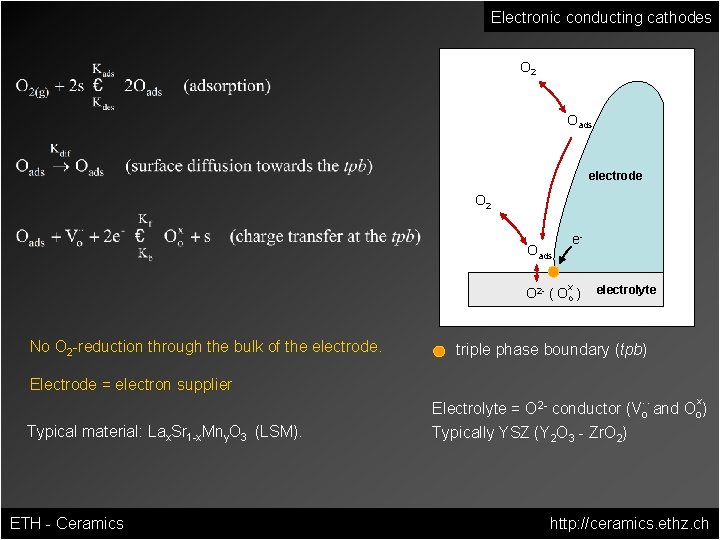
Electronic conducting cathodes O 2 Oads electrode O 2 e- Oads x O 2 - ( Oo ) No O 2 -reduction through the bulk of the electrode. Electrode = electron supplier Typical material: Lax. Sr 1 -x. Mny. O 3 (LSM). ETH - Ceramics electrolyte triple phase boundary (tpb) x. . Electrolyte = O 2 - conductor (Vo and Oo) Typically YSZ (Y 2 O 3 - Zr. O 2) http: //ceramics. ethz. ch
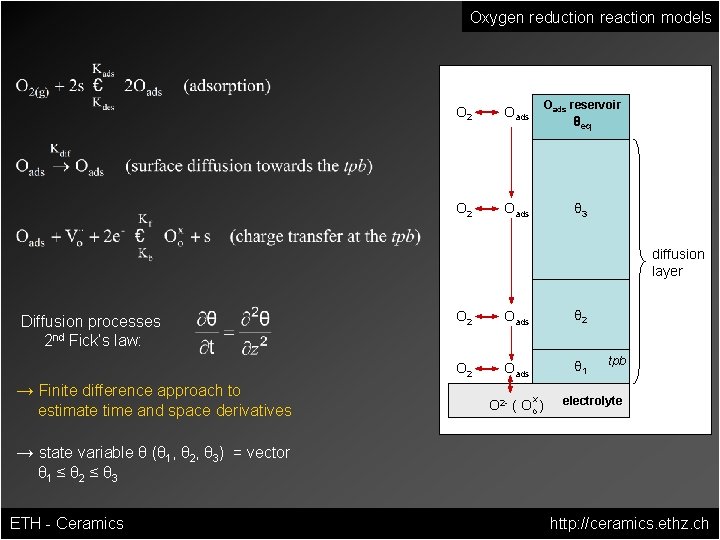
Oxygen reduction reaction models O 2 Oads reservoir O 2 Oads θ 3 θeq diffusion layer Diffusion processes 2 nd Fick‘s law: → Finite difference approach to estimate time and space derivatives O 2 Oads θ 1 x O 2 - ( Oo ) tpb electrolyte → state variable θ (θ 1, θ 2, θ 3) = vector θ 1 ≤ θ 2 ≤ θ 3 ETH - Ceramics http: //ceramics. ethz. ch
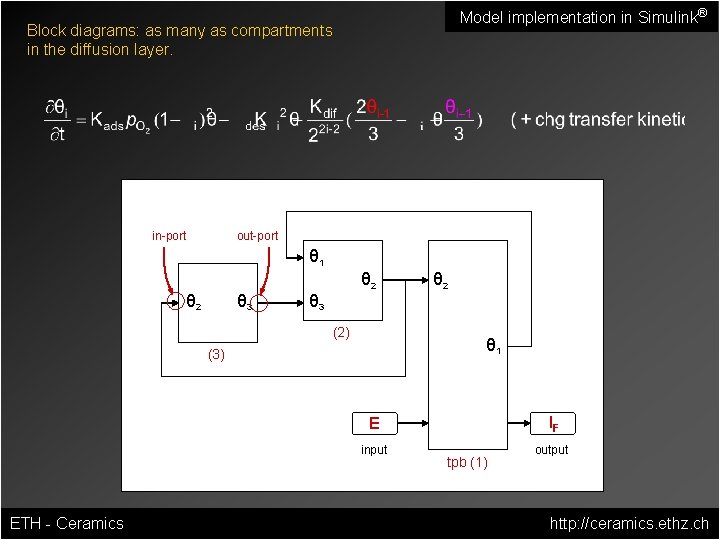
Model implementation in Simulink® Block diagrams: as many as compartments in the diffusion layer. in-port out-port θ 1 θ 2 θ 3 (2) θ 2 θ 1 (3) IF E input ETH - Ceramics tpb (1) output http: //ceramics. ethz. ch
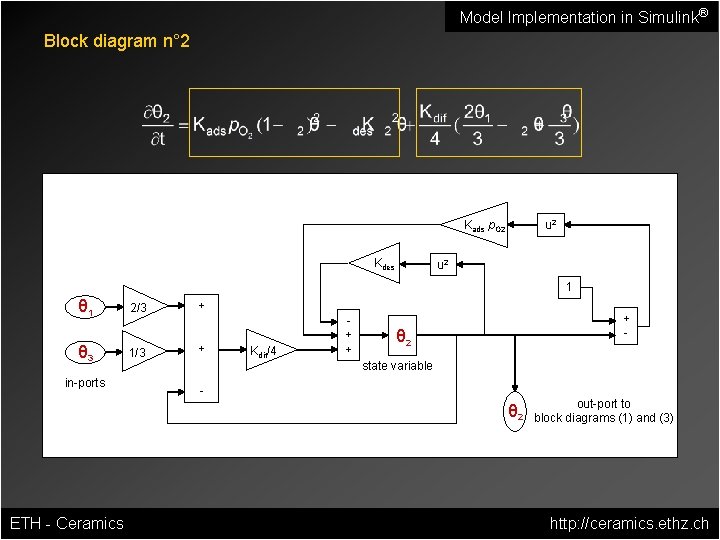
Model Implementation in Simulink® Block diagram n° 2 Kads p. O 2 Kdes u 2 1 Kdif/4 + + + - θ 2 state variable - in-ports 1/3 + θ 3 2/3 + θ 1 θ 2 ETH - Ceramics out-port to block diagrams (1) and (3) http: //ceramics. ethz. ch
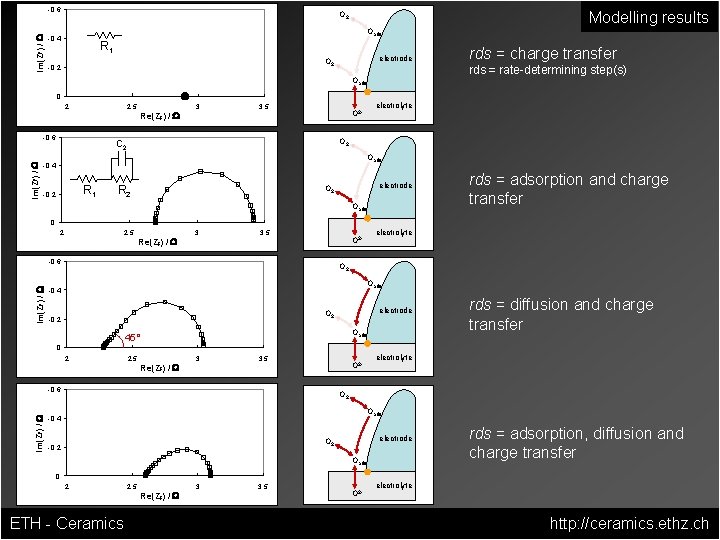
Im(ZF) / W -0. 6 Modelling results O 2 Oads -0. 4 R 1 electrode O 2 -0. 2 rds = charge transfer rds = rate-determining step(s) Oads 0 2 2. 5 Im(ZF) / W -0. 6 Re(ZF) / W 3 3. 5 O 2 - electrolyte O 2 C 2 Oads -0. 4 R 1 -0. 2 R 2 electrode O 2 Oads rds = adsorption and charge transfer 0 2 2. 5 Re(ZF) / W 3 3. 5 O 2 - Im(ZF) / W -0. 6 electrolyte O 2 Oads -0. 4 electrode O 2 -0. 2 Oads 45° rds = diffusion and charge transfer 0 2 2. 5 Re(ZF) / W 3 3. 5 O 2 - Im(ZF) / W -0. 6 electrolyte O 2 Oads -0. 4 electrode O 2 -0. 2 Oads rds = adsorption, diffusion and charge transfer 0 2 ETH - Ceramics 2. 5 Re(ZF) / W 3 3. 5 O 2 - electrolyte http: //ceramics. ethz. ch
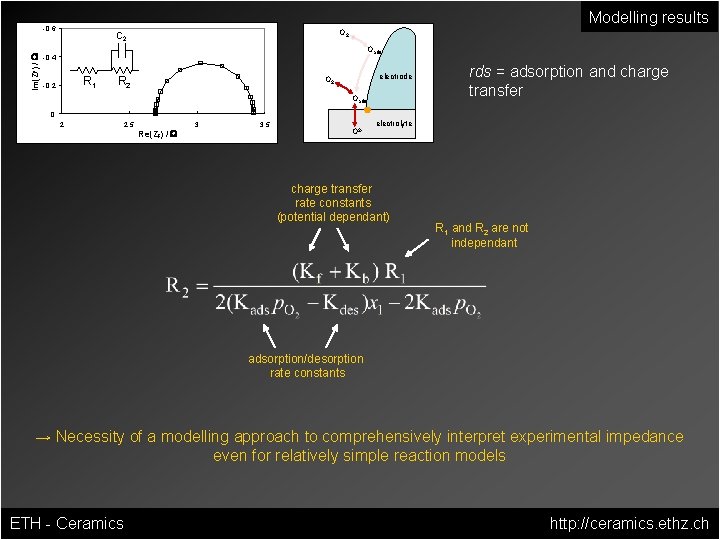
Modelling results Im(ZF) / W -0. 6 O 2 C 2 Oads -0. 4 R 1 -0. 2 R 2 electrode O 2 Oads rds = adsorption and charge transfer 0 2 2. 5 Re(ZF) / W 3 3. 5 O 2 - electrolyte charge transfer rate constants (potential dependant) R 1 and R 2 are not independant adsorption/desorption rate constants → Necessity of a modelling approach to comprehensively interpret experimental impedance even for relatively simple reaction models ETH - Ceramics http: //ceramics. ethz. ch
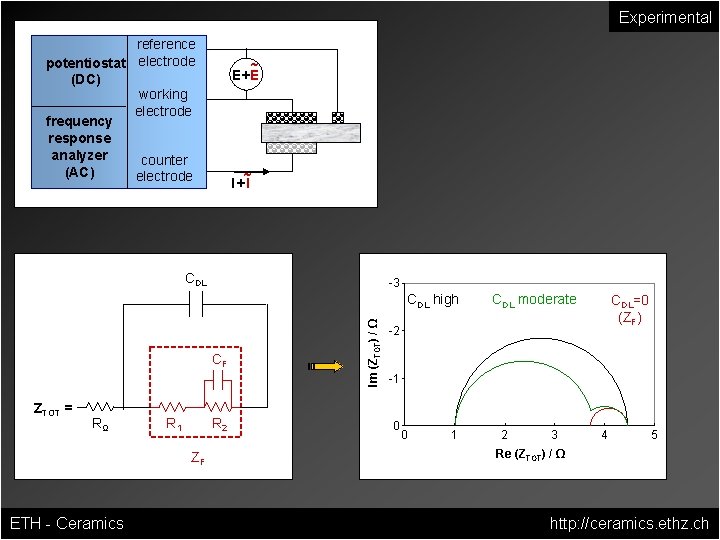
Experimental reference potentiostat electrode (DC) working electrode frequency response analyzer counter (AC) electrode ~ E+E ~ I+I CDL -3 CF ZTOT = RΩ R 1 R 2 ZF ETH - Ceramics Im (ZTOT) / Ω CDL high CDL moderate CDL=0 (ZF) -2 -1 0 0 1 2 3 4 5 Re (ZTOT) / Ω http: //ceramics. ethz. ch
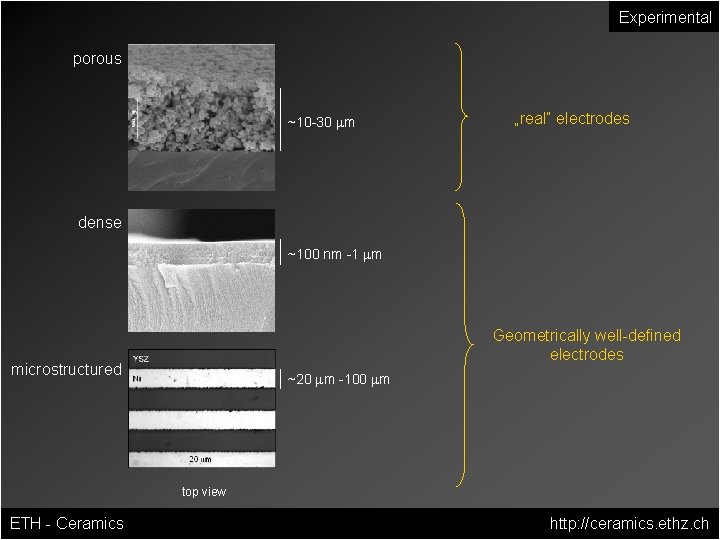
Experimental porous ~10 -30 mm „real“ electrodes dense ~100 nm -1 mm Geometrically well-defined electrodes microstructured ~20 mm -100 mm top view ETH - Ceramics http: //ceramics. ethz. ch
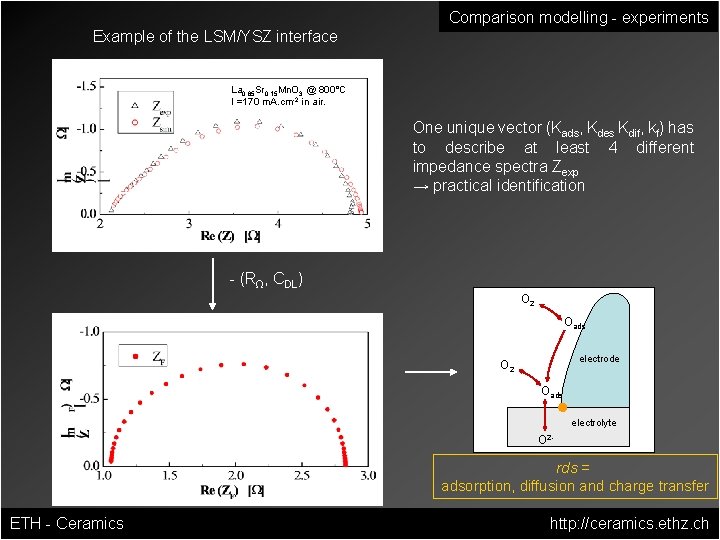
Comparison modelling - experiments Example of the LSM/YSZ interface La 0. 85 Sr 0. 15 Mn. O 3 @ 800°C I =170 m. A. cm-2 in air. One unique vector (Kads, Kdes Kdif, kf) has to describe at least 4 different impedance spectra Zexp → practical identification - (RΩ, CDL) O 2 Oads electrode O 2 Oads electrolyte O 2 - rds = adsorption, diffusion and charge transfer ETH - Ceramics http: //ceramics. ethz. ch
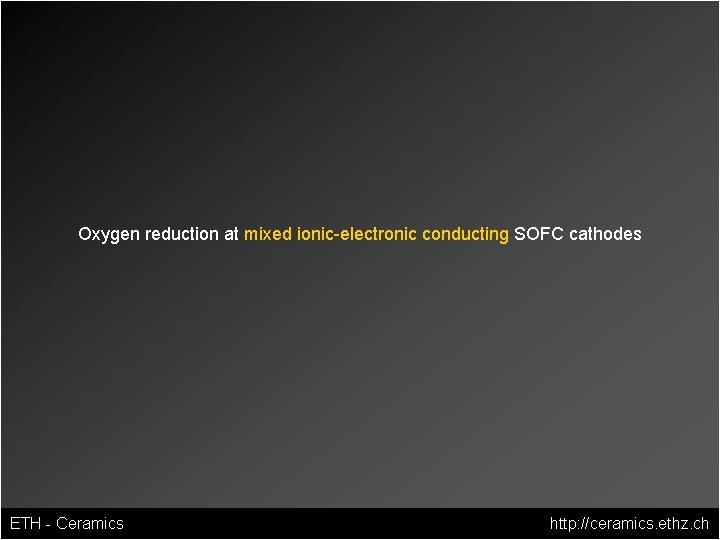
Oxygen reduction at mixed ionic-electronic conducting SOFC cathodes ETH - Ceramics http: //ceramics. ethz. ch
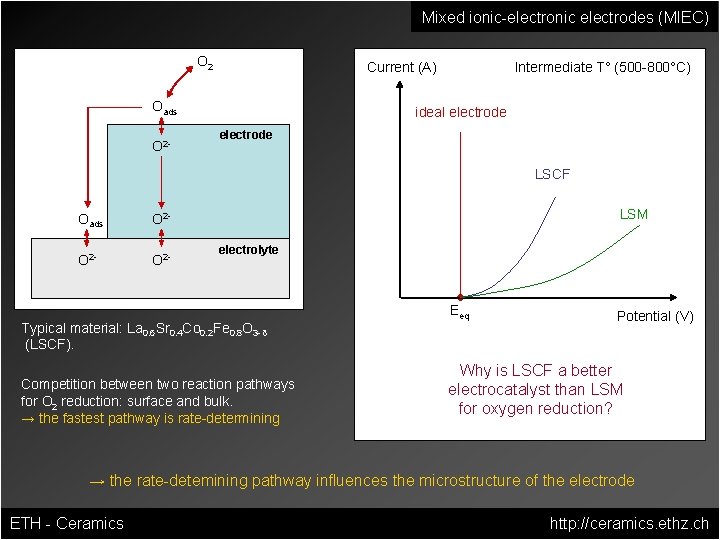
Mixed ionic-electronic electrodes (MIEC) O 2 Current (A) Oads O 2 - Intermediate T° (500 -800°C) ideal electrode LSCF Oads O 2 - LSM electrolyte Typical material: La 0. 6 Sr 0. 4 Co 0. 2 Fe 0. 8 O 3 -δ (LSCF). Competition between two reaction pathways for O 2 reduction: surface and bulk. → the fastest pathway is rate-determining Eeq Potential (V) Why is LSCF a better electrocatalyst than LSM for oxygen reduction? → the rate-detemining pathway influences the microstructure of the electrode ETH - Ceramics http: //ceramics. ethz. ch
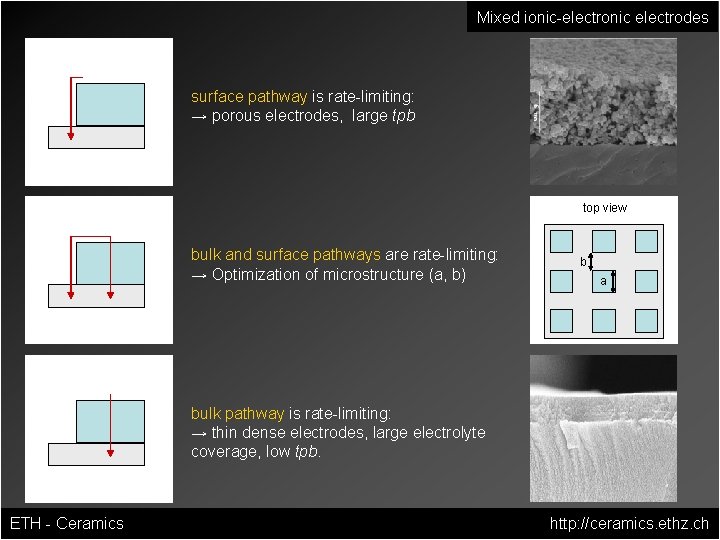
Mixed ionic-electronic electrodes surface pathway is rate-limiting: → porous electrodes, large tpb top view bulk and surface pathways are rate-limiting: → Optimization of microstructure (a, b) b a bulk pathway is rate-limiting: → thin dense electrodes, large electrolyte coverage, low tpb. ETH - Ceramics http: //ceramics. ethz. ch
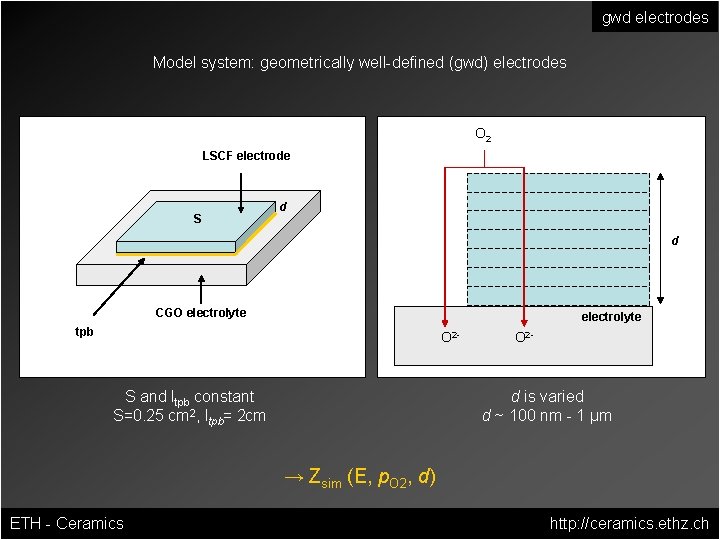
gwd electrodes Model system: geometrically well-defined (gwd) electrodes O 2 LSCF electrode S d d CGO electrolyte tpb O 2 - S and ltpb constant S=0. 25 cm 2, ltpb= 2 cm O 2 - d is varied d ~ 100 nm - 1 µm → Zsim (E, p. O 2, d) ETH - Ceramics http: //ceramics. ethz. ch
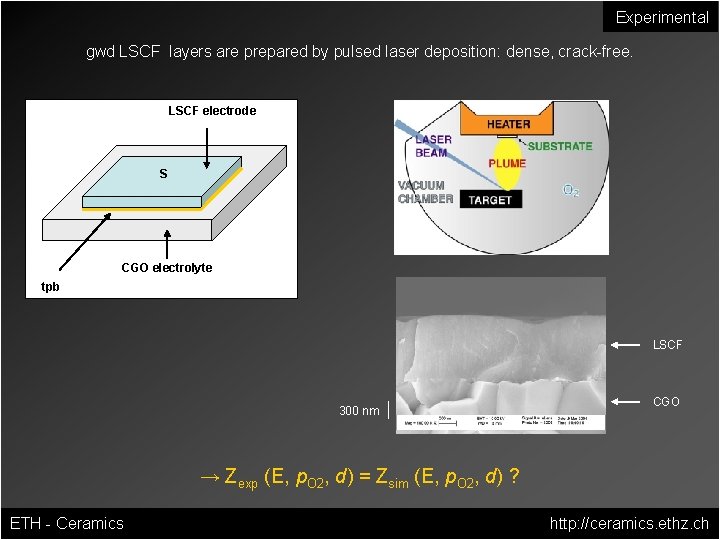
Experimental gwd LSCF layers are prepared by pulsed laser deposition: dense, crack-free. LSCF electrode S CGO electrolyte tpb LSCF 300 nm CGO → Zexp (E, p. O 2, d) = Zsim (E, p. O 2, d) ? ETH - Ceramics http: //ceramics. ethz. ch
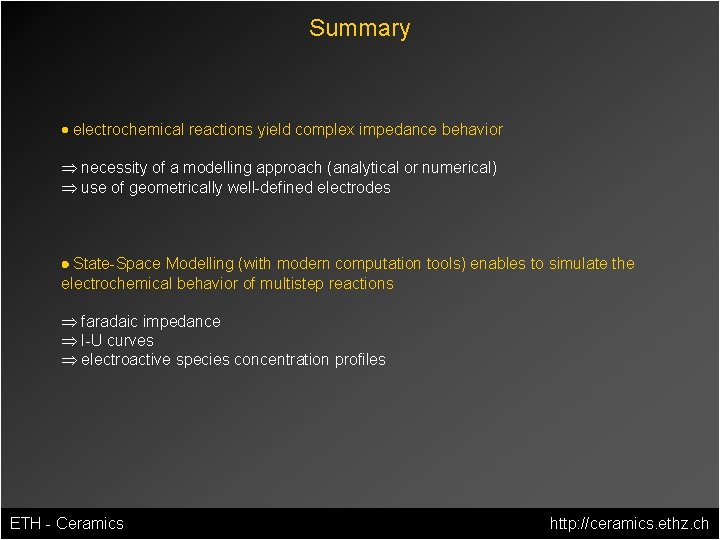
Summary · electrochemical reactions yield complex impedance behavior Þ necessity of a modelling approach (analytical or numerical) Þ use of geometrically well-defined electrodes · State-Space Modelling (with modern computation tools) enables to simulate the electrochemical behavior of multistep reactions Þ faradaic impedance Þ I-U curves Þ electroactive species concentration profiles ETH - Ceramics http: //ceramics. ethz. ch
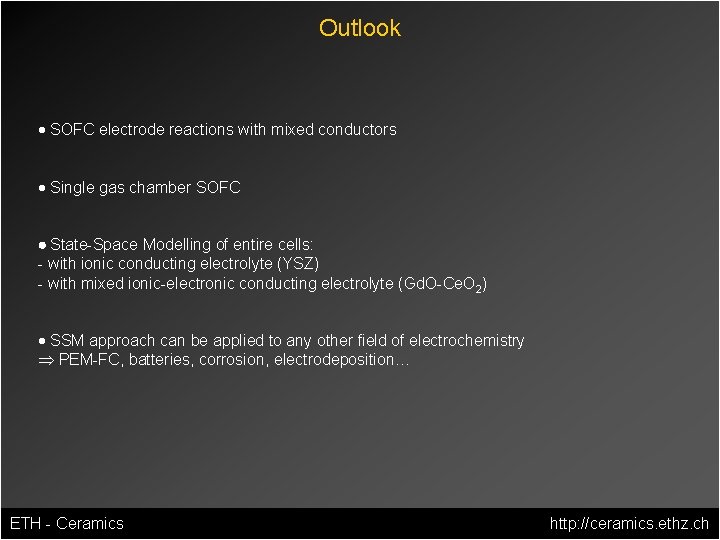
Outlook · SOFC electrode reactions with mixed conductors · Single gas chamber SOFC · State-Space Modelling of entire cells: - with ionic conducting electrolyte (YSZ) - with mixed ionic-electronic conducting electrolyte (Gd. O-Ce. O 2) · SSM approach can be applied to any other field of electrochemistry Þ PEM-FC, batteries, corrosion, electrodeposition… ETH - Ceramics http: //ceramics. ethz. ch
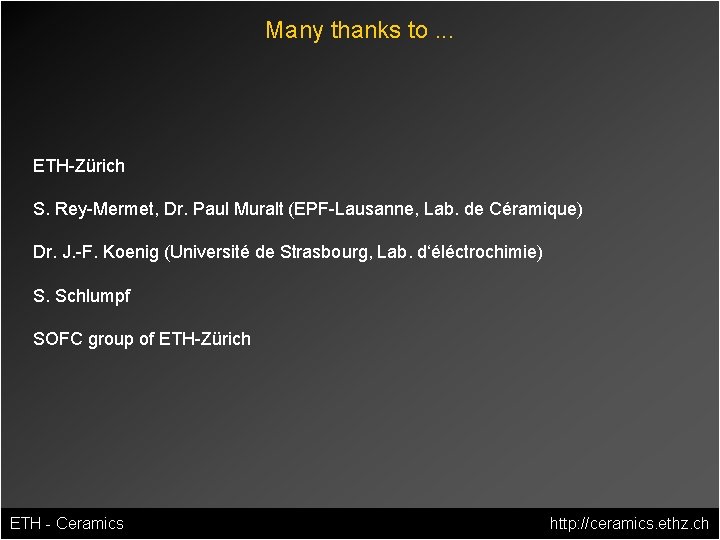
Many thanks to. . . ETH-Zürich S. Rey-Mermet, Dr. Paul Muralt (EPF-Lausanne, Lab. de Céramique) Dr. J. -F. Koenig (Université de Strasbourg, Lab. d‘éléctrochimie) S. Schlumpf SOFC group of ETH-Zürich ETH - Ceramics http: //ceramics. ethz. ch
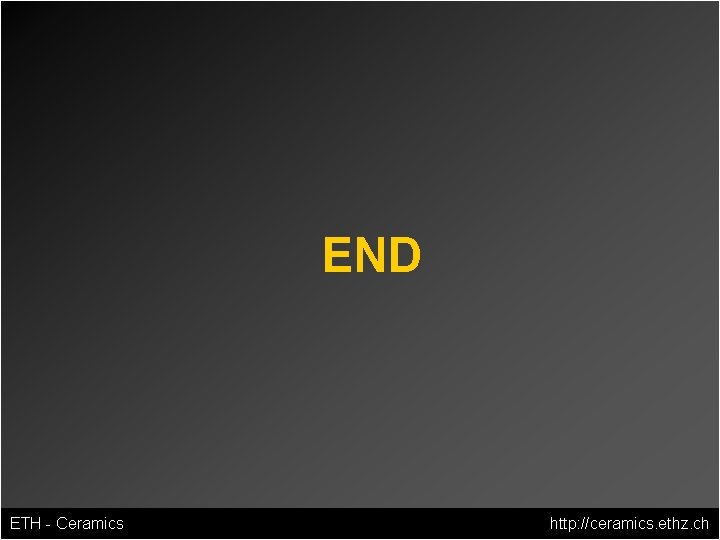
END ETH - Ceramics http: //ceramics. ethz. ch
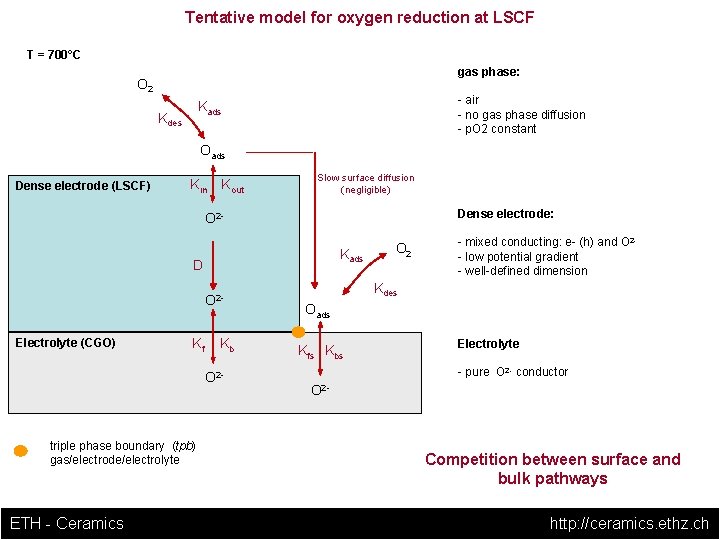
Tentative model for oxygen reduction at LSCF T = 700°C gas phase: O 2 - air - no gas phase diffusion - p. O 2 constant Kads Kdes Oads Dense electrode (LSCF) Kin Kout Slow surface diffusion (negligible) Dense electrode: O 2 Kads D O 2 Electrolyte (CGO) Kf Kb O 2 - triple phase boundary (tpb) gas/electrode/electrolyte ETH - Ceramics O 2 - mixed conducting: e- (h) and O 2 - low potential gradient - well-defined dimension Kdes Oads Kfs Kbs Electrolyte - pure O 2 - conductor O 2 - Competition between surface and bulk pathways http: //ceramics. ethz. ch
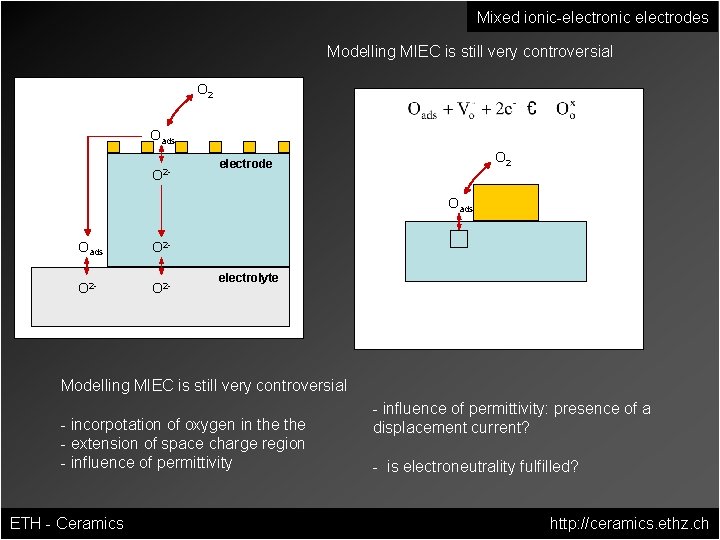
Mixed ionic-electronic electrodes Modelling MIEC is still very controversial O 2 Oads O 2 - O 2 electrode Oads O 2 - electrolyte Modelling MIEC is still very controversial - incorpotation of oxygen in the - extension of space charge region - influence of permittivity ETH - Ceramics - influence of permittivity: presence of a displacement current? - is electroneutrality fulfilled? http: //ceramics. ethz. ch
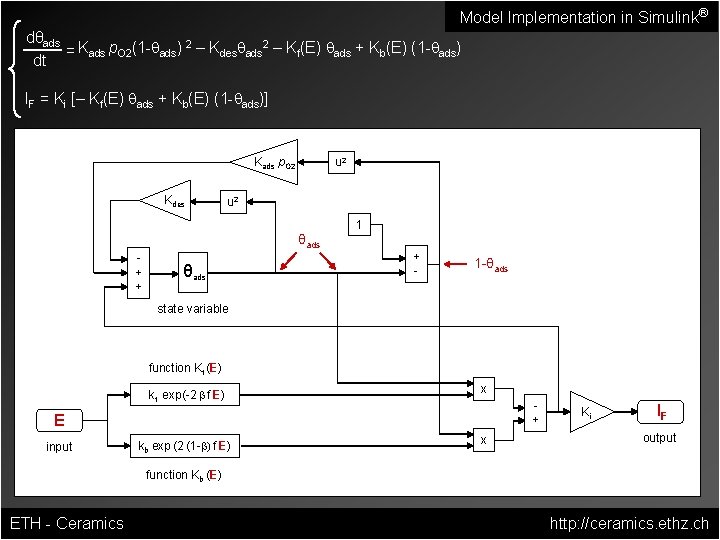
Model Implementation in Simulink® dθads 2 2 = Kads p. O 2(1 -qads) – Kdesqads – Kf(E) qads + Kb(E) (1 -qads) dt IF = Ki [– Kf(E) qads + Kb(E) (1 -qads)] Kads p. O 2 Kdes u 2 1 θads + + θads + - 1 -θads state variable function Kf (E) kf exp(-2 b f E) x + E input kb exp (2 (1 -b) f E) x Ki IF output function Kb (E) ETH - Ceramics http: //ceramics. ethz. ch
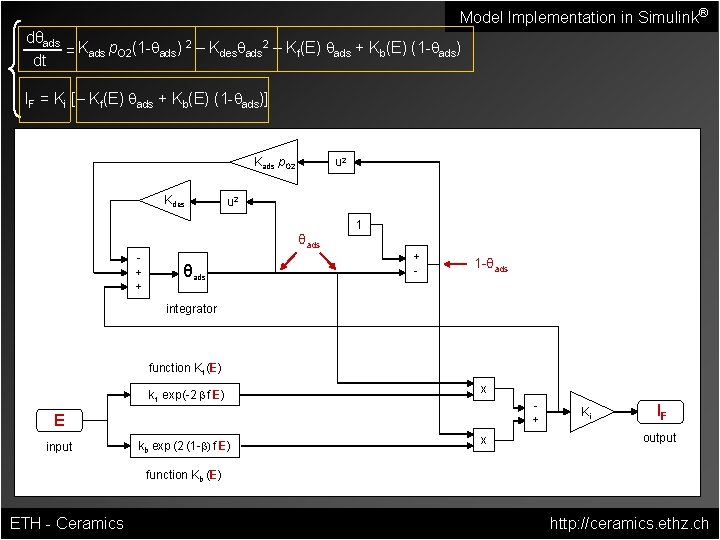
Model Implementation in Simulink® dθads 2 2 = Kads p. O 2(1 -qads) – Kdesqads – Kf(E) qads + Kb(E) (1 -qads) dt IF = Ki [– Kf(E) qads + Kb(E) (1 -qads)] Kads p. O 2 Kdes u 2 1 θads + + θads + - 1 -θads integrator function Kf (E) kf exp(-2 b f E) x + E input kb exp (2 (1 -b) f E) x Ki IF output function Kb (E) ETH - Ceramics http: //ceramics. ethz. ch
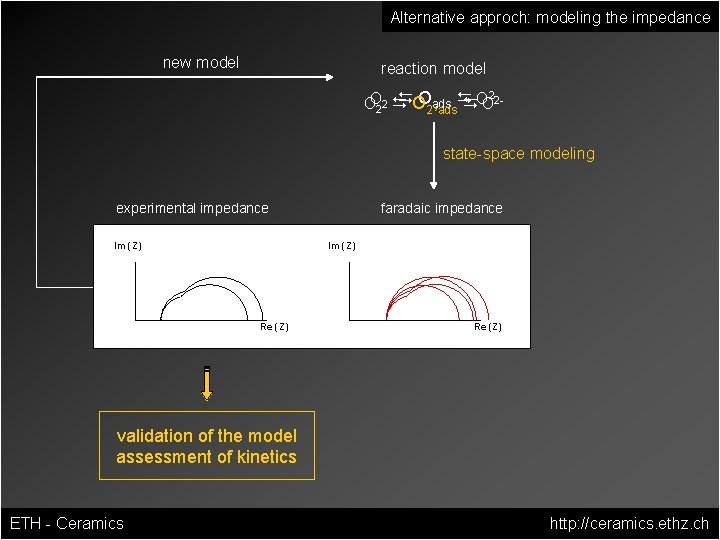
Alternative approch: modeling the impedance new model reaction model DOOads D OO 2 -2 OO 22 D , D 2 ads state-space modeling experimental impedance Im (Z) faradaic impedance Im (Z) Re (Z) validation of the model assessment of kinetics ETH - Ceramics http: //ceramics. ethz. ch
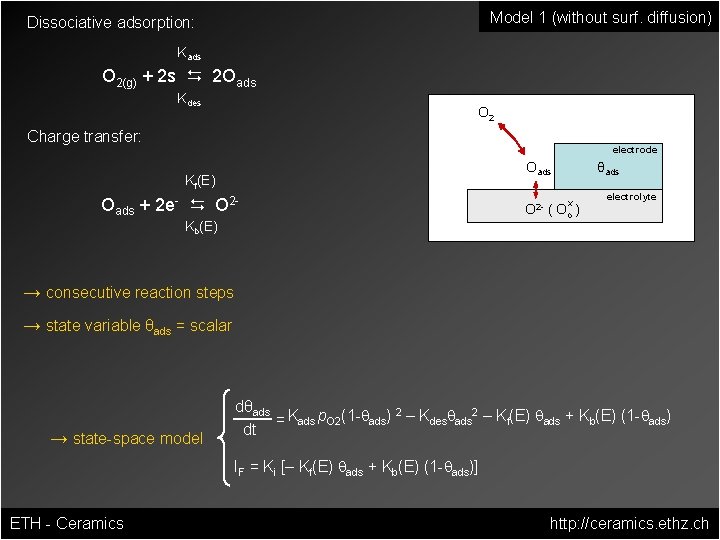
Model 1 (without surf. diffusion) Dissociative adsorption: Kads O 2(g) + 2 s D 2 Oads Kdes O 2 Charge transfer: electrode Oads Kf(E) Oads + 2 e- D O 2 Kb(E) θads x O 2 - ( Oo ) electrolyte → consecutive reaction steps → state variable θads = scalar → state-space model dθads 2 2 = Kads p. O 2(1 -qads) – Kdesqads – Kf(E) qads + Kb(E) (1 -qads) dt IF = Ki [– Kf(E) qads + Kb(E) (1 -qads)] ETH - Ceramics http: //ceramics. ethz. ch
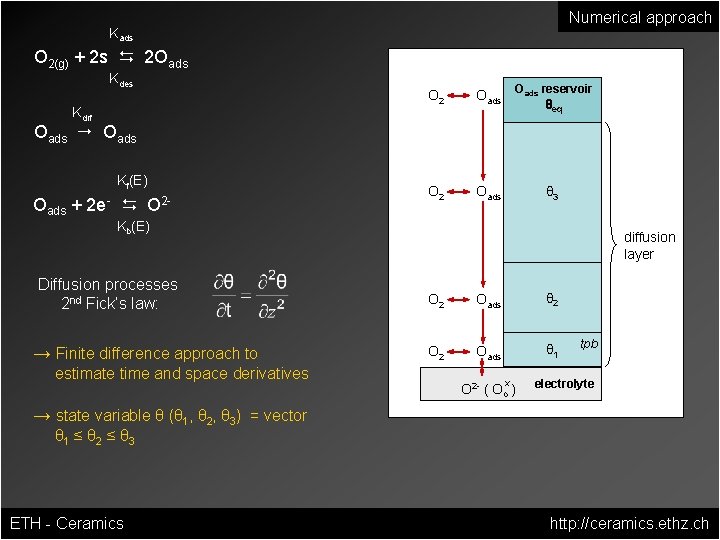
Numerical approach Kads O 2(g) + 2 s D 2 Oads Kdes Kdif O 2 Oads reservoir O 2 Oads θ 3 θeq Oads " Oads Kf(E) Oads + 2 e- D O 2 Kb(E) Diffusion processes 2 nd Fick‘s law: → Finite difference approach to estimate time and space derivatives diffusion layer O 2 Oads θ 1 x O 2 - ( Oo ) tpb electrolyte → state variable θ (θ 1, θ 2, θ 3) = vector θ 1 ≤ θ 2 ≤ θ 3 ETH - Ceramics http: //ceramics. ethz. ch
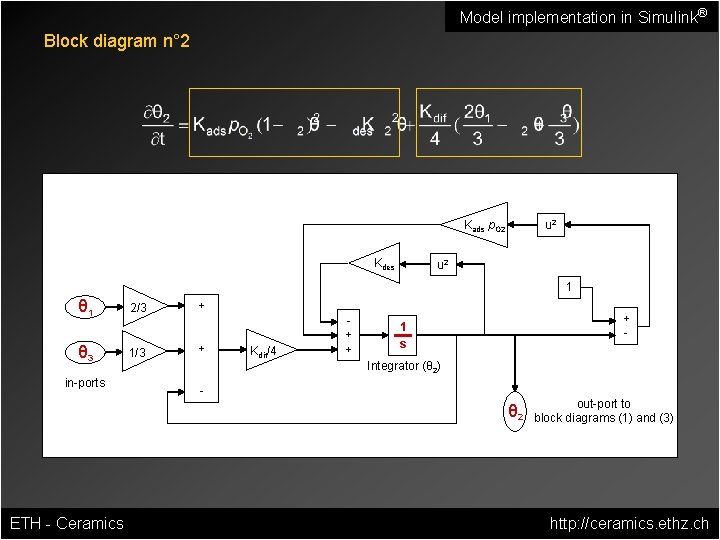
Model implementation in Simulink® Block diagram n° 2 Kads p. O 2 Kdes u 2 1 Kdif/4 + + + - 1 s Integrator (θ 2) - in-ports 1/3 + θ 3 2/3 + θ 1 θ 2 ETH - Ceramics out-port to block diagrams (1) and (3) http: //ceramics. ethz. ch
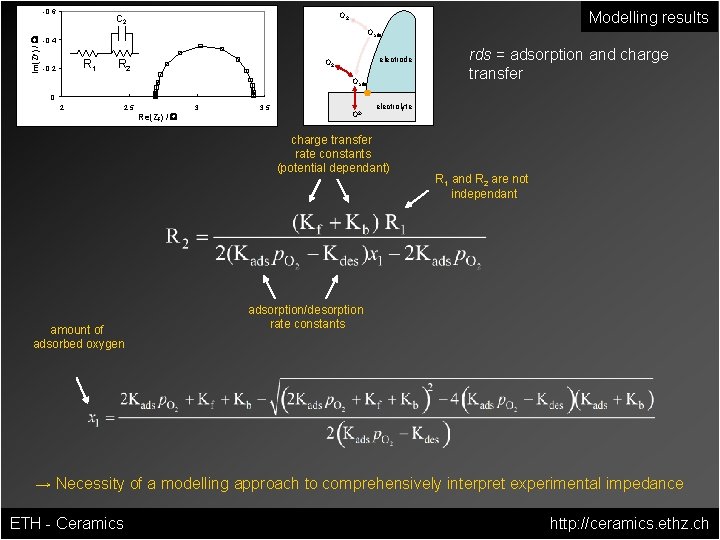
Im(ZF) / W -0. 6 Modelling results O 2 C 2 Oads -0. 4 R 1 -0. 2 R 2 electrode O 2 Oads rds = adsorption and charge transfer 0 2 2. 5 Re(ZF) / W 3 3. 5 O 2 - electrolyte charge transfer rate constants (potential dependant) amount of adsorbed oxygen R 1 and R 2 are not independant adsorption/desorption rate constants → Necessity of a modelling approach to comprehensively interpret experimental impedance ETH - Ceramics http: //ceramics. ethz. ch
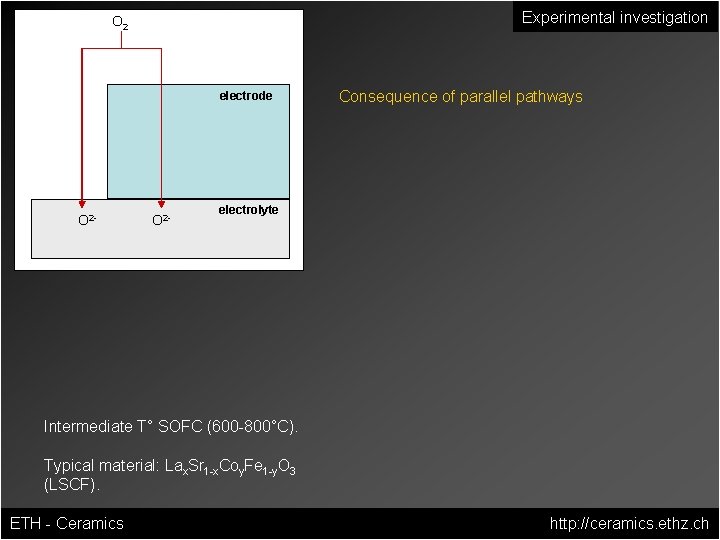
Experimental investigation O 2 electrode O 2 - Consequence of parallel pathways electrolyte Intermediate T° SOFC (600 -800°C). Typical material: Lax. Sr 1 -x. Coy. Fe 1 -y. O 3 (LSCF). ETH - Ceramics http: //ceramics. ethz. ch
Steam cycle
Sofc
Sofc
Central pocket whorl vs plain whorl
Ngt the talk chapter 6
3 electrode
Esp electrodes
Biopotential electrodes lecture notes
Electrolysis of sulphuric acid
Aps therapy electrodes
Swd frequency wavelength
Fhc electrodes
Hittorfs law
Floating electrodes for ecg
Spoon shaped electrode
Fcaw electrodes
Diathermy diagram
Hamilton sus
Logam karbon
Floating electrodes for ecg
Biopotential electrodes
Electrodes ecg
Flat position welding
Biopotential electrodes
Types of indicator electrode in potentiometry
Half time equation
Rate of reaction formula
Addition reaction and substitution reaction
Leukoerythroblastic reaction vs leukemoid reaction
The three steps of polymerase chain reaction
What is axial movement in dance
Livestock identification methods
Isa electrical symbols
Immunofluorescence
Tentative layout
Project identification process
Aircraft wire identification