High energy neutrino astronomy What we have learned
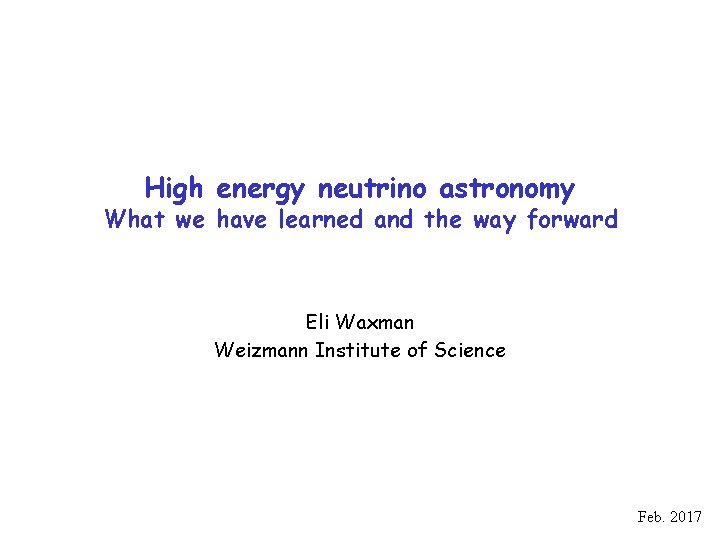
![log [dn/d. E] The main driver of HE n astronomy: The origin of Cosmic log [dn/d. E] The main driver of HE n astronomy: The origin of Cosmic](https://slidetodoc.com/presentation_image_h2/4f19c03c5bfdd3bd203e523f60186e16/image-2.jpg)
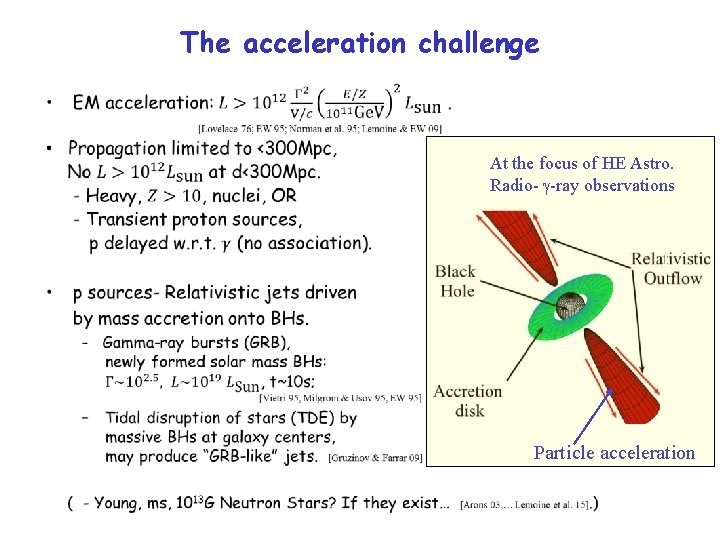
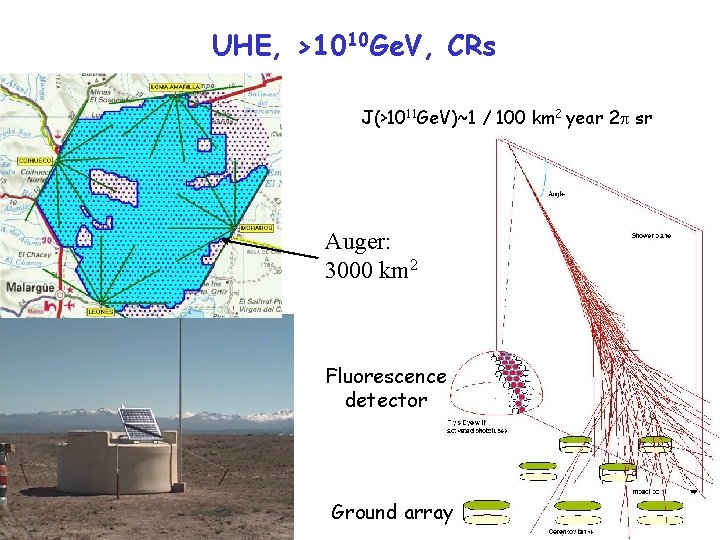
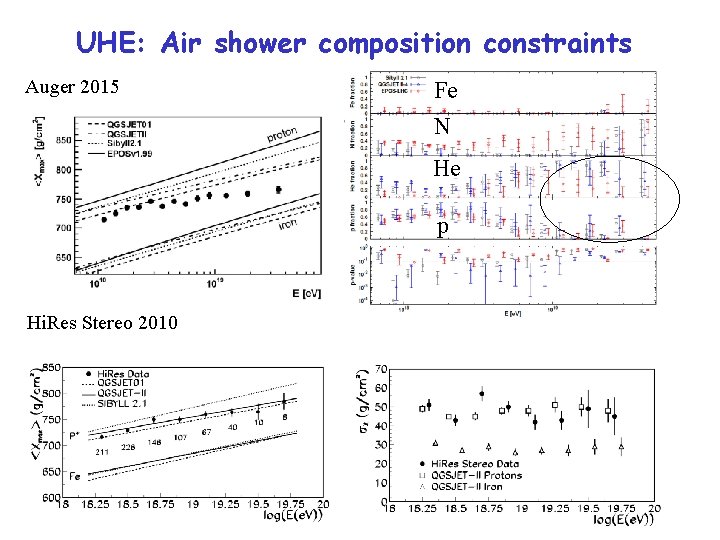
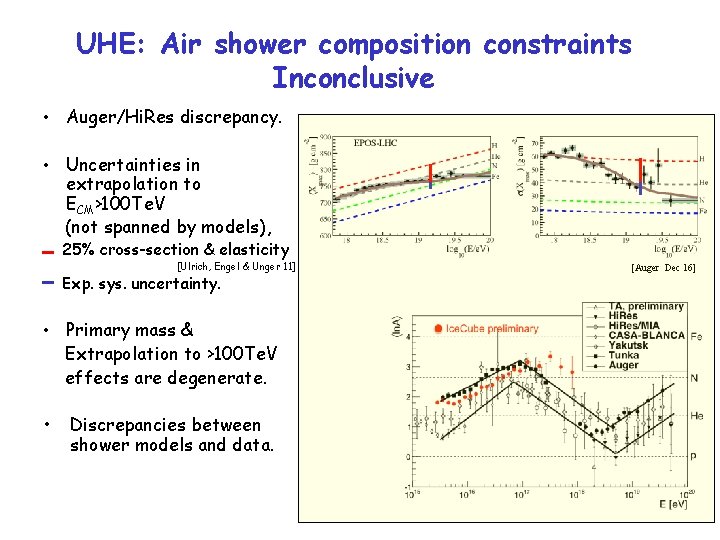
![>1010 Ge. V spectrum: a hint to p’s cteff [Mpc] GZK >1010 Ge. V spectrum: a hint to p’s cteff [Mpc] GZK](https://slidetodoc.com/presentation_image_h2/4f19c03c5bfdd3bd203e523f60186e16/image-7.jpg)
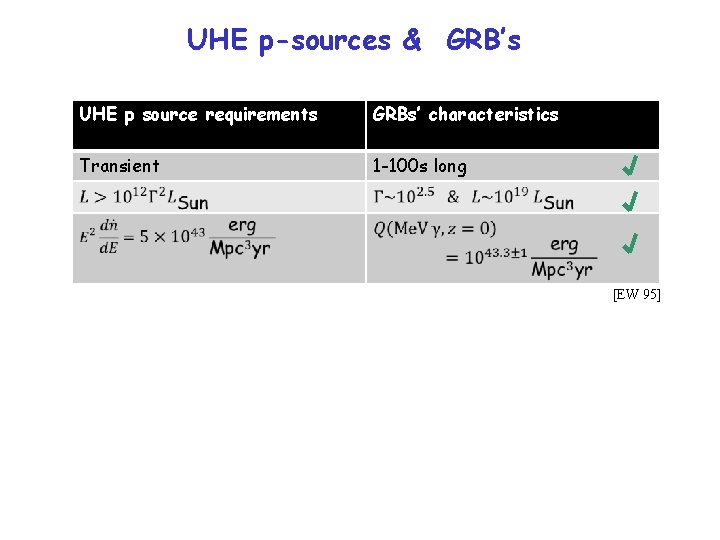
![A mixed composition? N p He Fe [Auger Dec 16] A mixed composition? N p He Fe [Auger Dec 16]](https://slidetodoc.com/presentation_image_h2/4f19c03c5bfdd3bd203e523f60186e16/image-9.jpg)
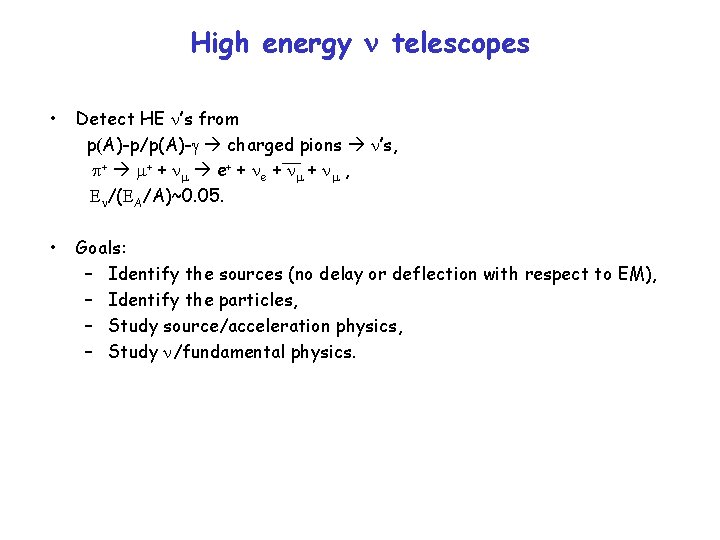
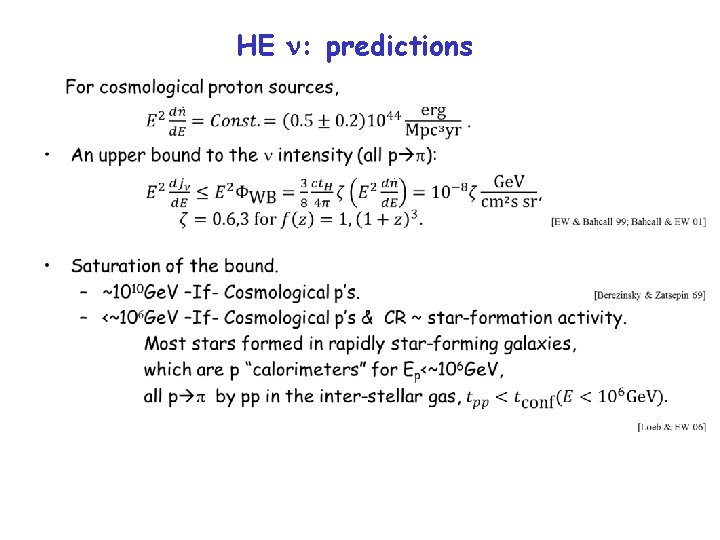
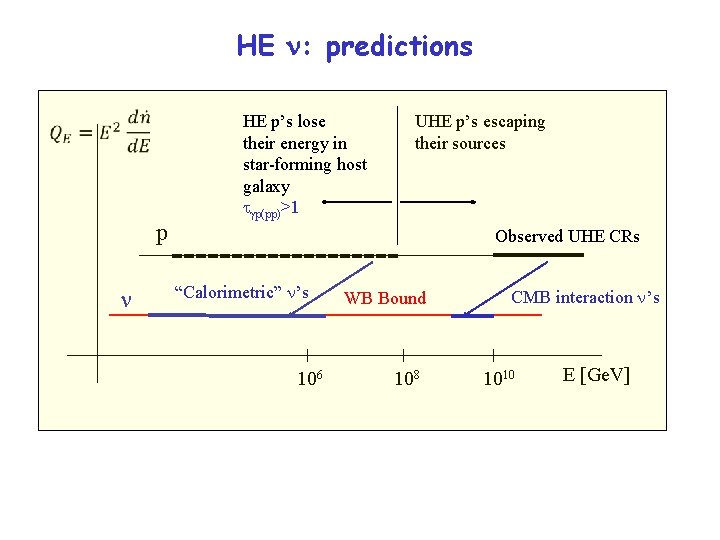
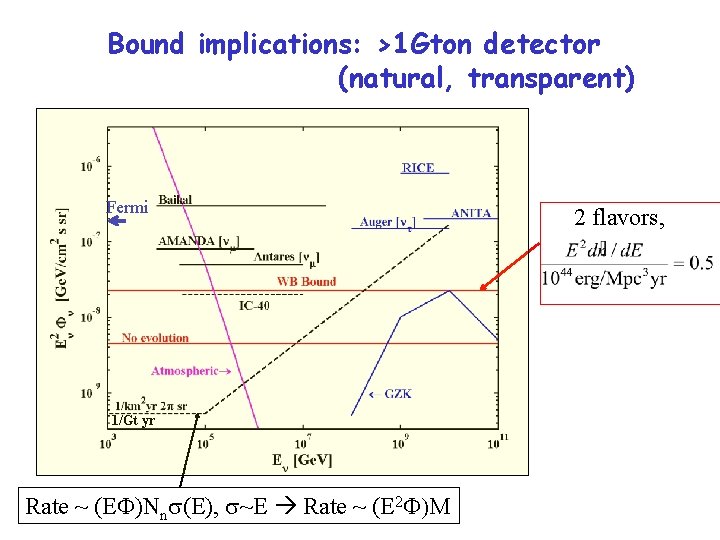
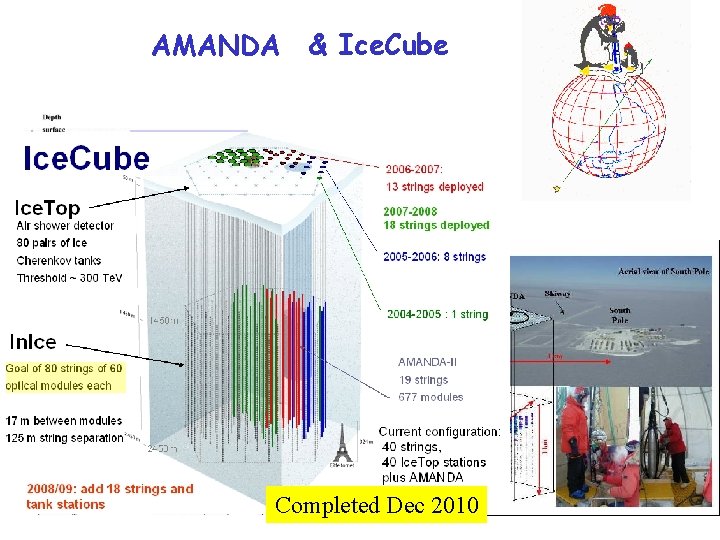
![Looking up: Vetoing atmospheric neutrinos [Schoenert, Gaisser et. al 2009] • Look for: Events Looking up: Vetoing atmospheric neutrinos [Schoenert, Gaisser et. al 2009] • Look for: Events](https://slidetodoc.com/presentation_image_h2/4f19c03c5bfdd3bd203e523f60186e16/image-15.jpg)
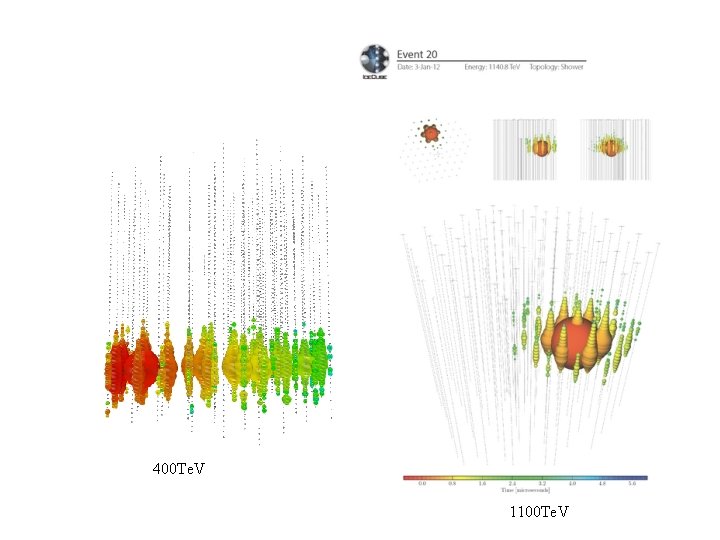
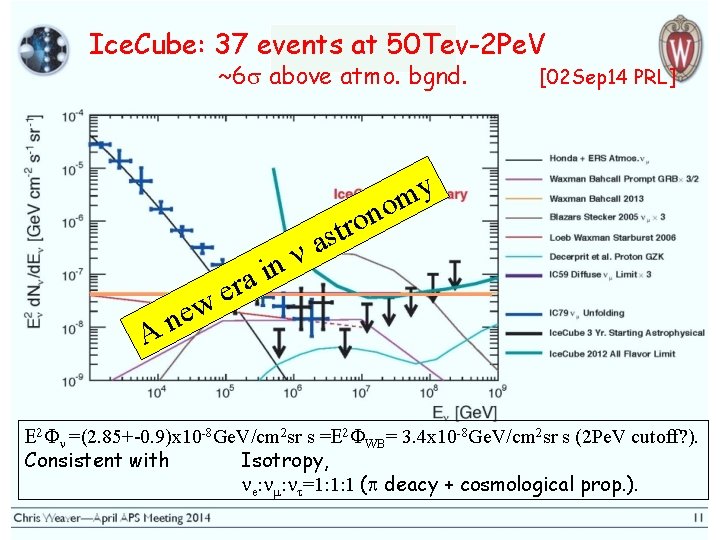
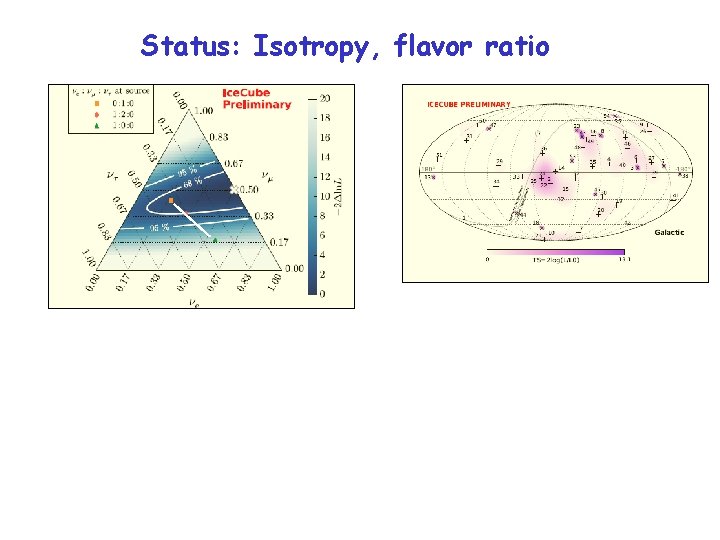
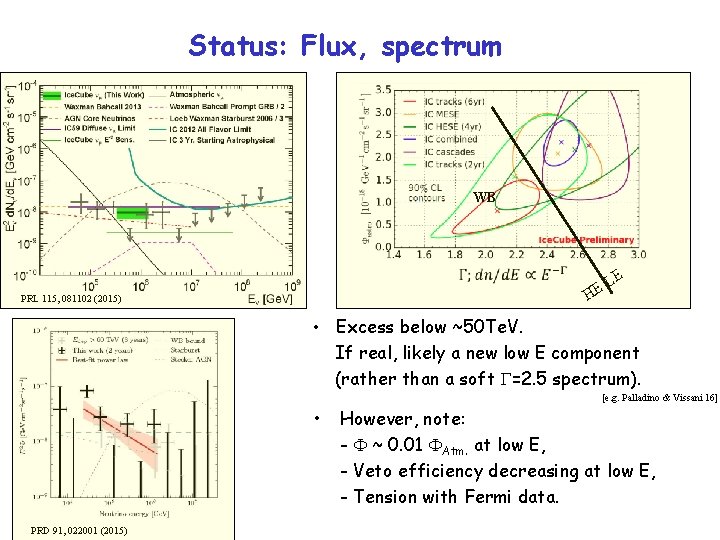
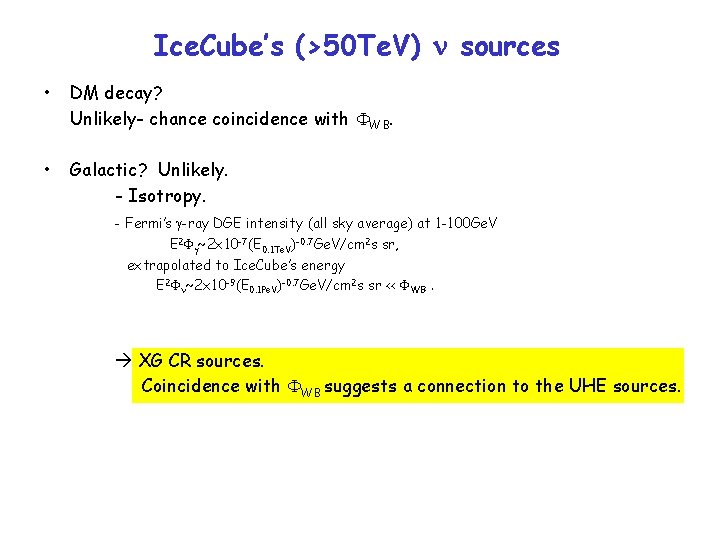
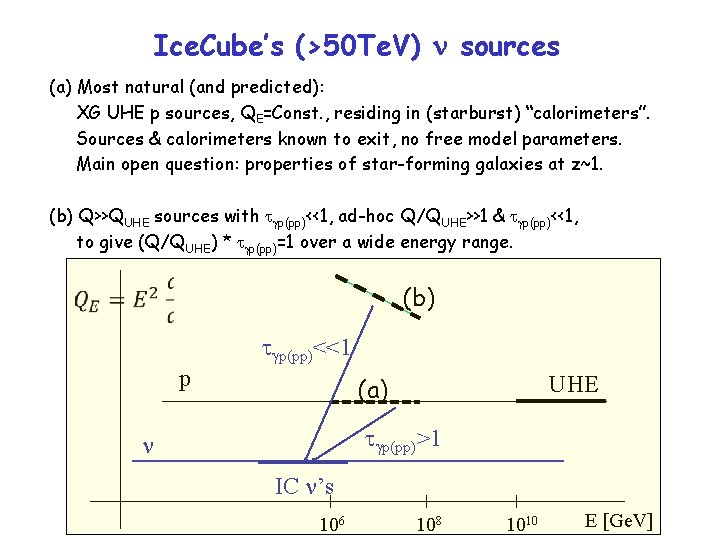
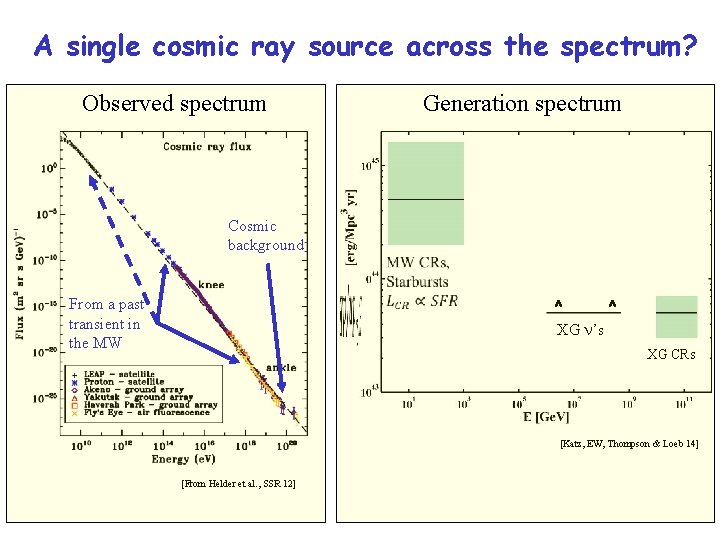
![Fermi’s XG g-ray background [EGB] • [Murase & EW 16] Murase 14 Fermi’s XG g-ray background [EGB] • [Murase & EW 16] Murase 14](https://slidetodoc.com/presentation_image_h2/4f19c03c5bfdd3bd203e523f60186e16/image-23.jpg)
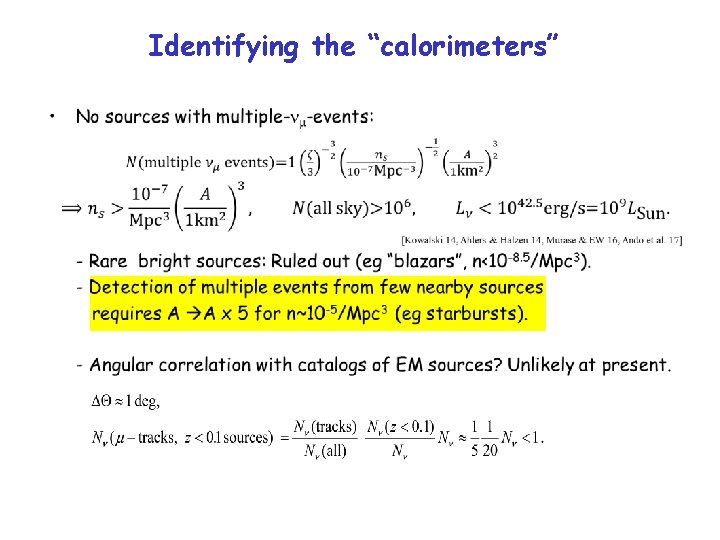
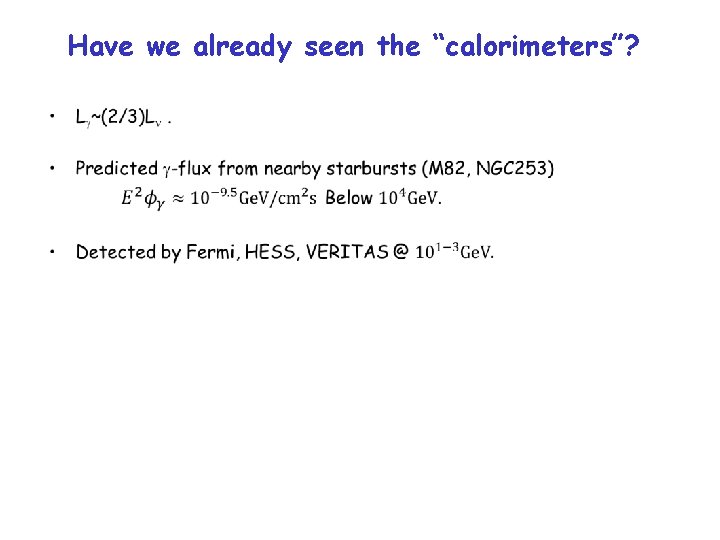
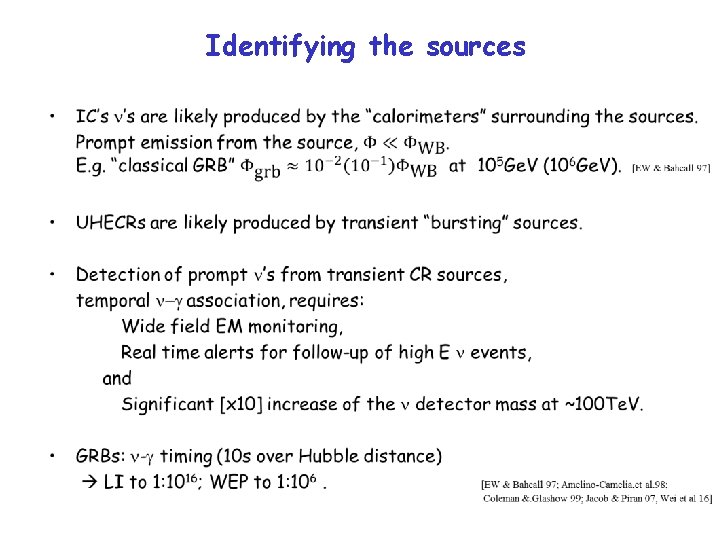
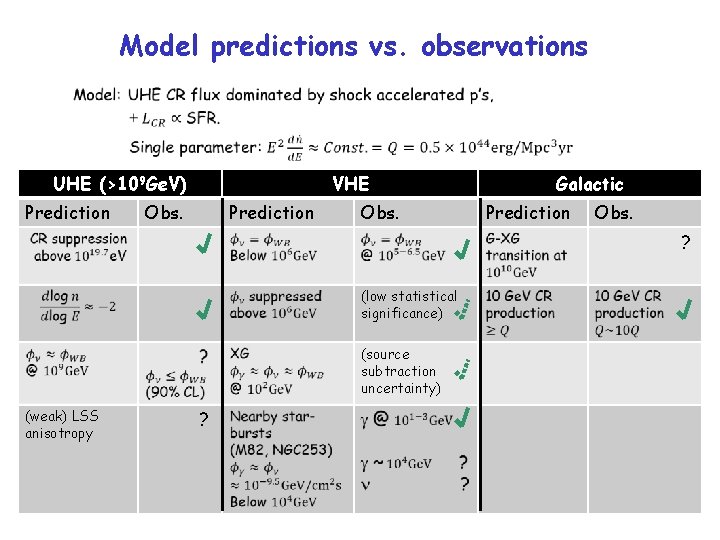
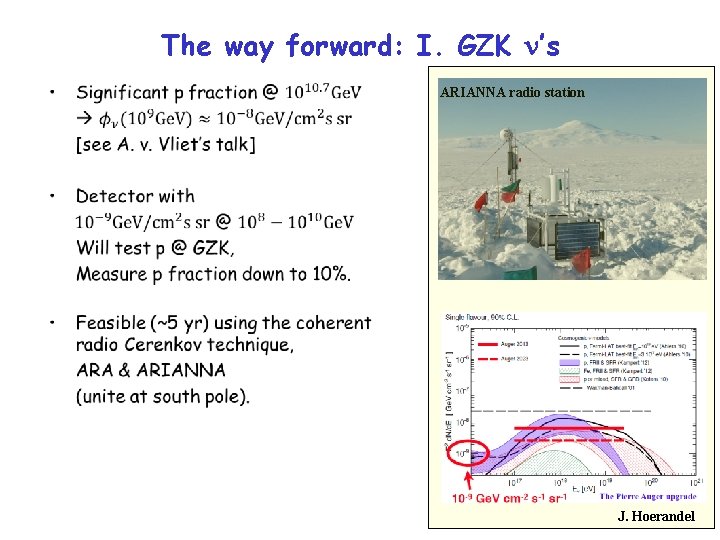
![Auger’s UHE limit [<2013/6 data] Auger’s UHE limit [<2013/6 data]](https://slidetodoc.com/presentation_image_h2/4f19c03c5bfdd3bd203e523f60186e16/image-29.jpg)
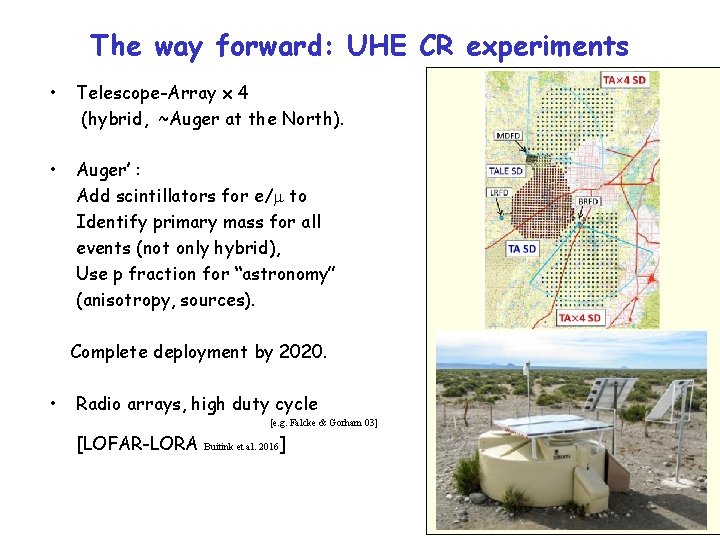
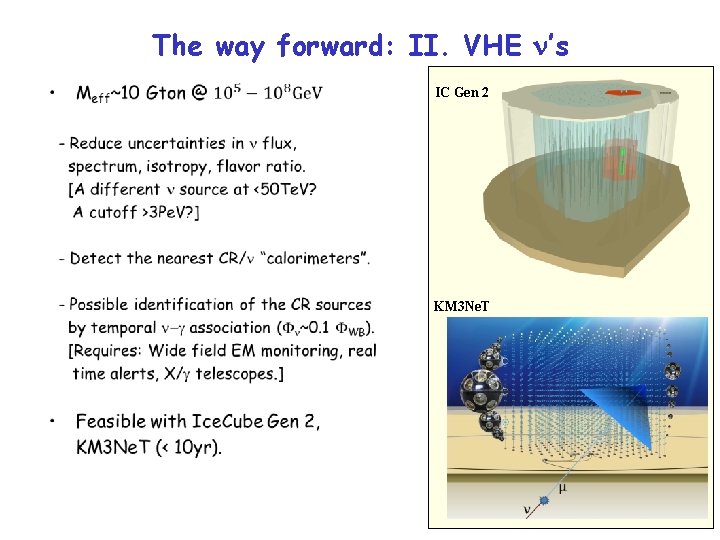
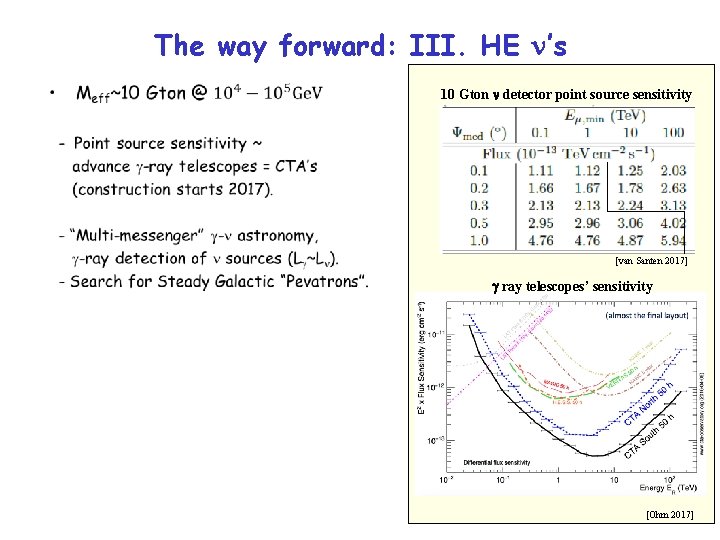
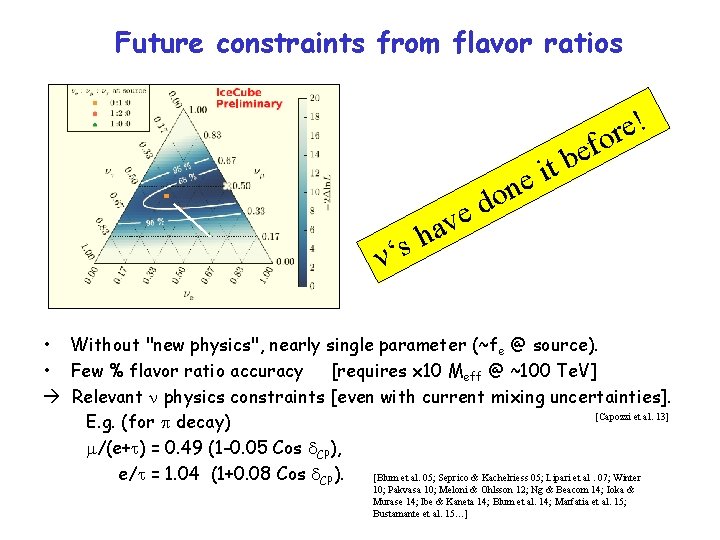
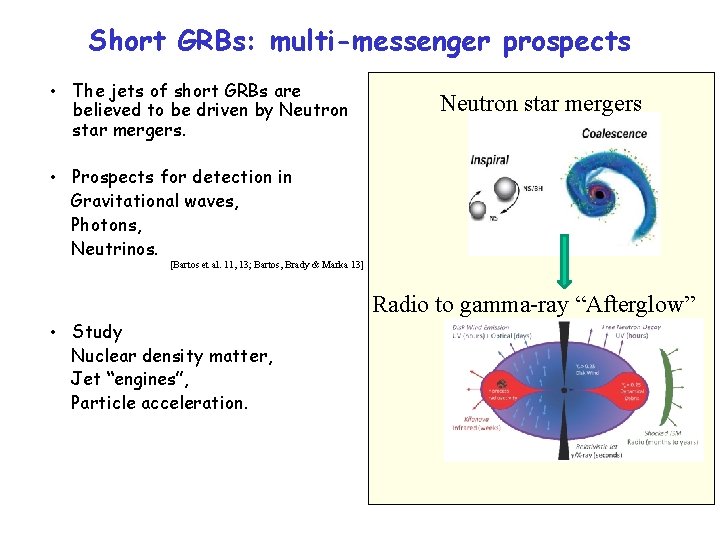
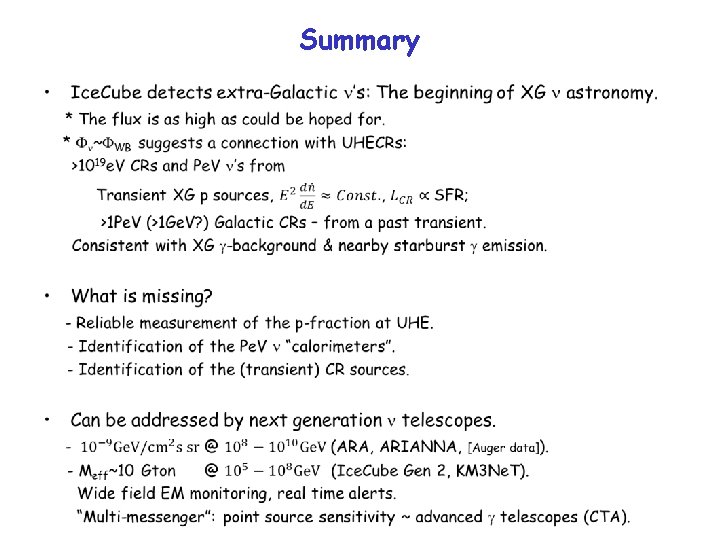
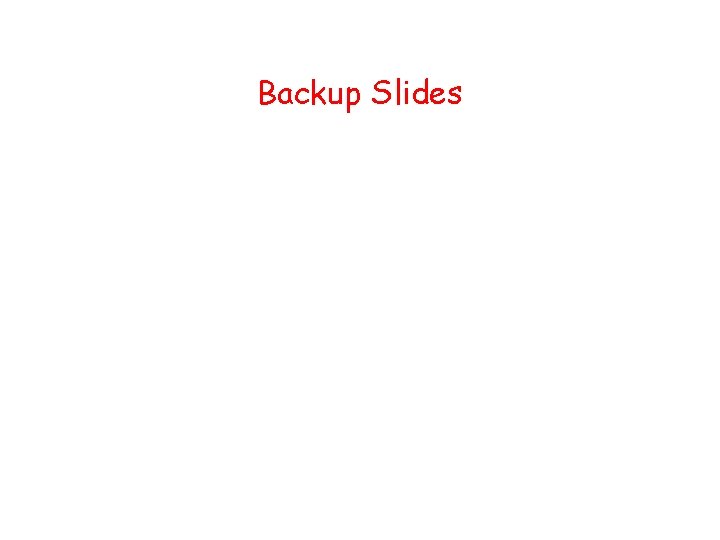
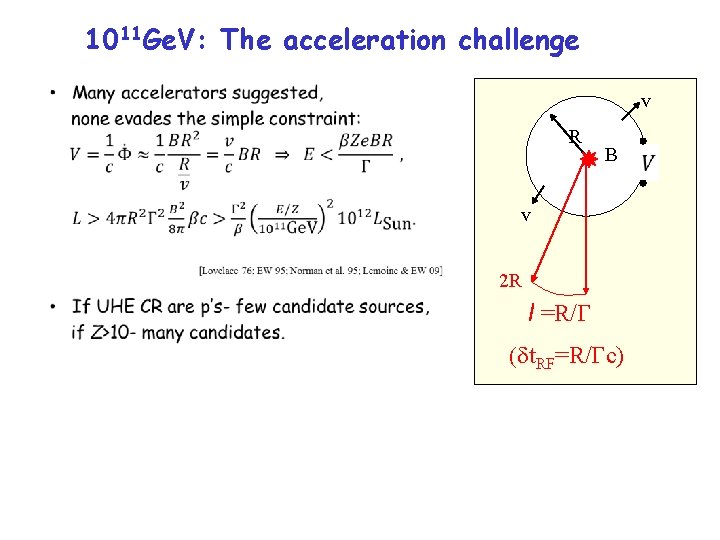
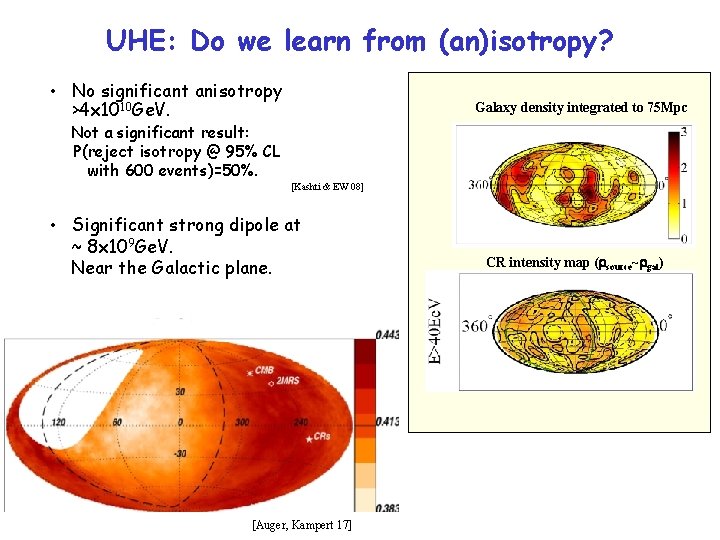
![A note on prompt GRB n’s • In [EW & Bahcall 97] WB 10% A note on prompt GRB n’s • In [EW & Bahcall 97] WB 10%](https://slidetodoc.com/presentation_image_h2/4f19c03c5bfdd3bd203e523f60186e16/image-39.jpg)
![Where is the G-XG transition? [Katz & EW 09] Where is the G-XG transition? [Katz & EW 09]](https://slidetodoc.com/presentation_image_h2/4f19c03c5bfdd3bd203e523f60186e16/image-40.jpg)
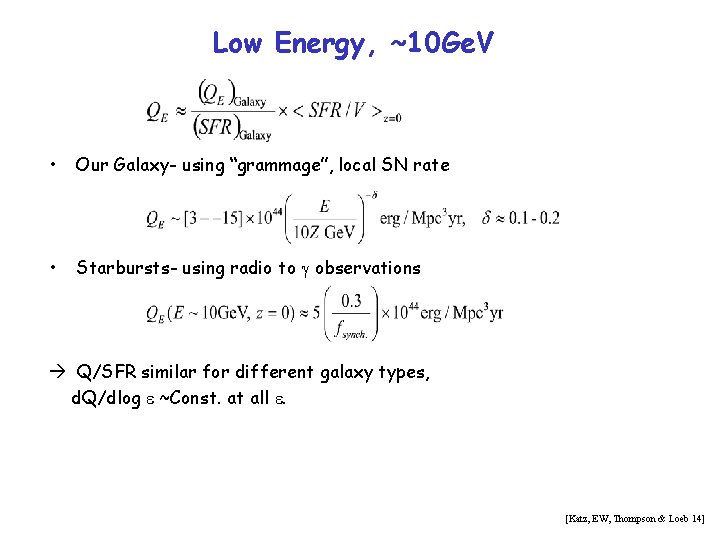
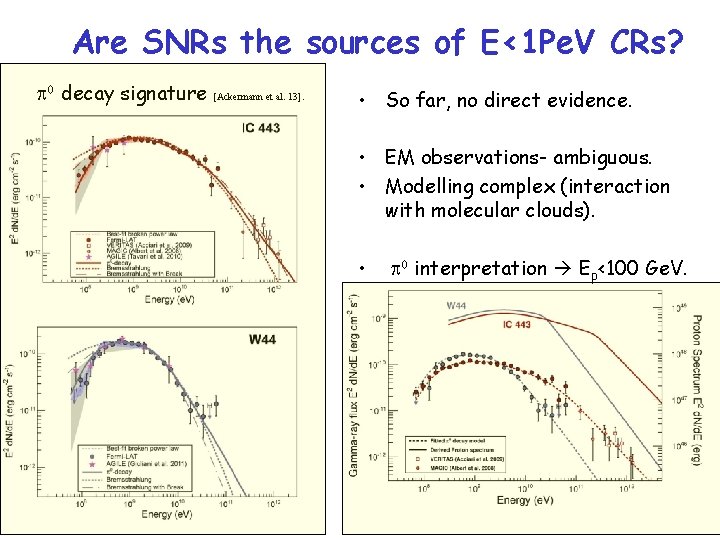
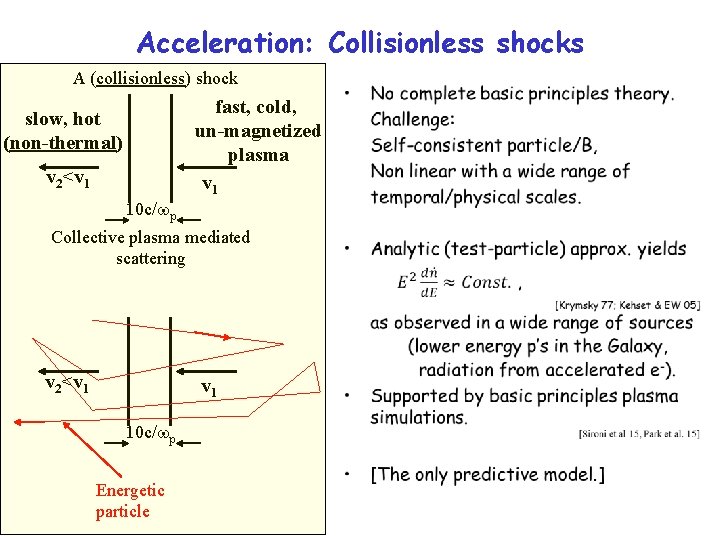
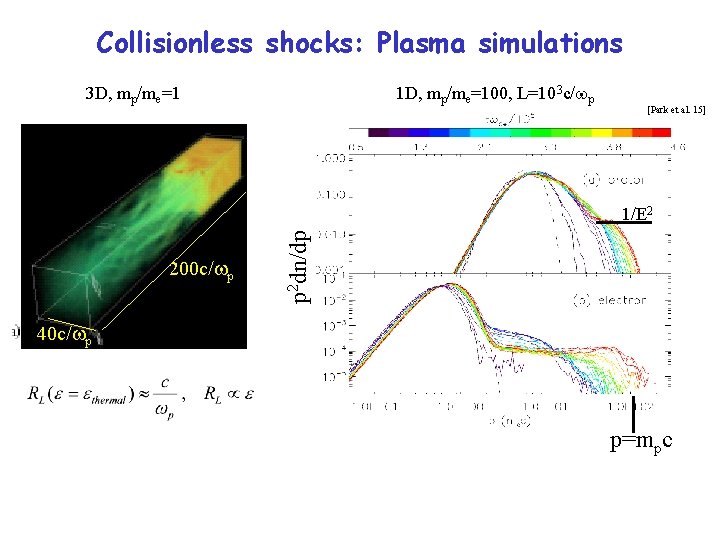
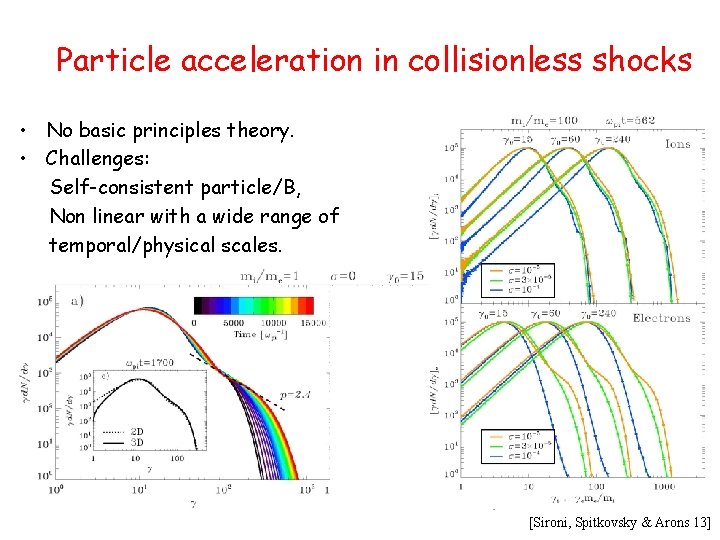
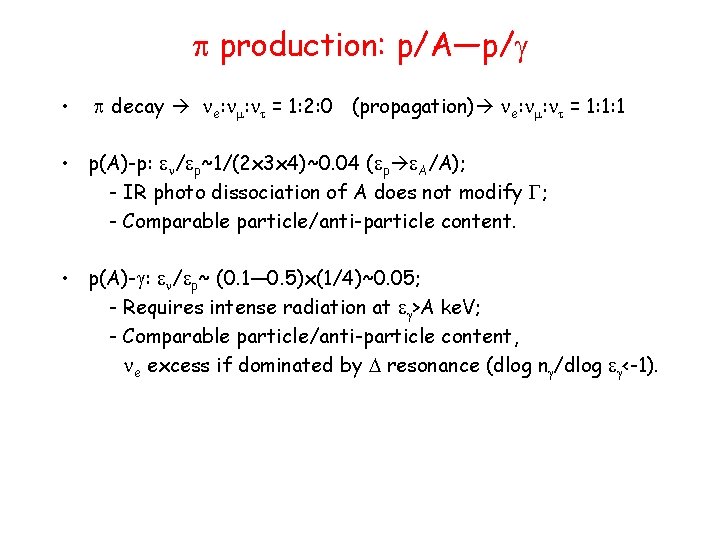
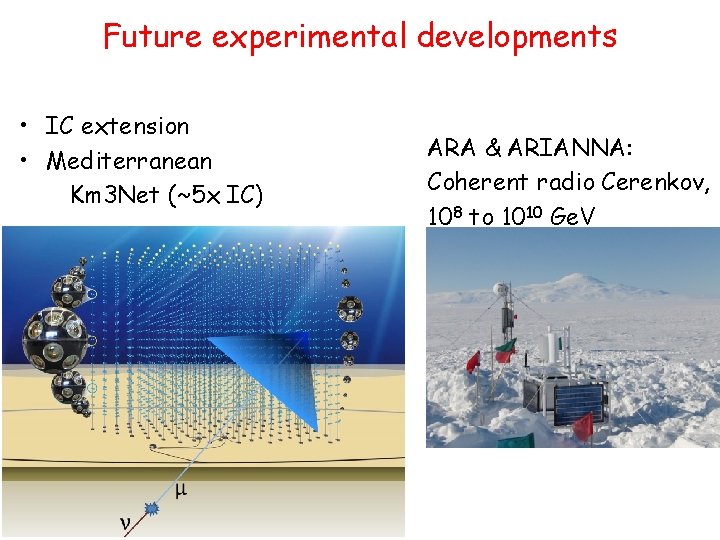
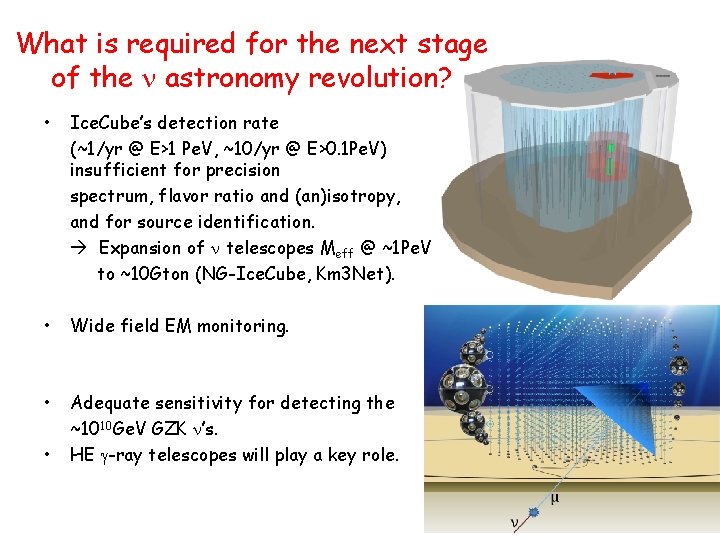
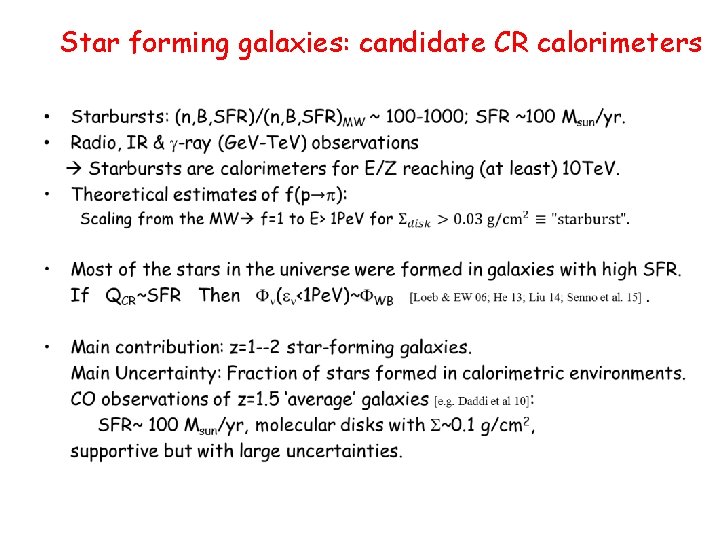
![[Rev. Sci. Inst. ] [Rev. Sci. Inst. ]](https://slidetodoc.com/presentation_image_h2/4f19c03c5bfdd3bd203e523f60186e16/image-50.jpg)
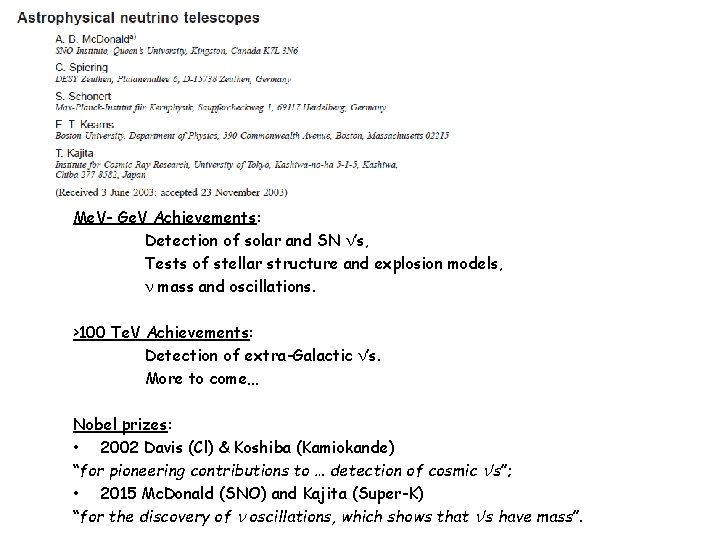
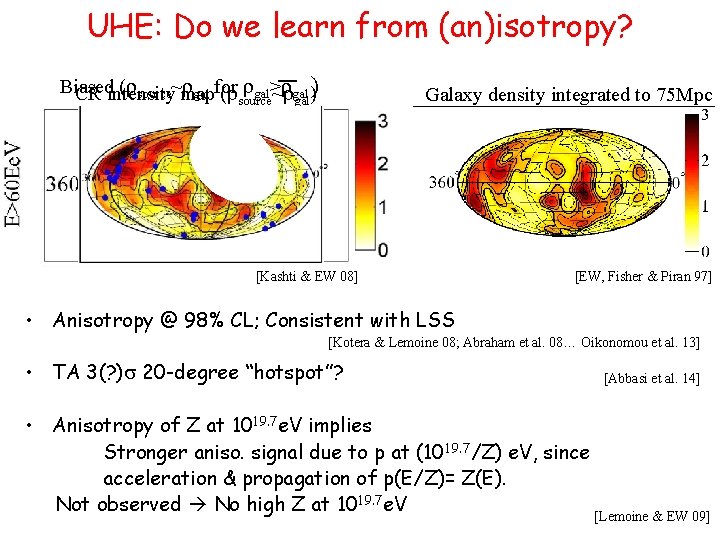
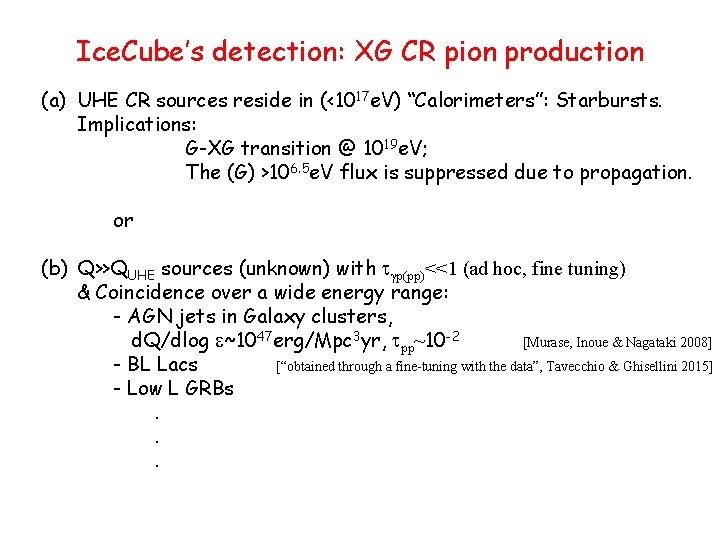
- Slides: 53
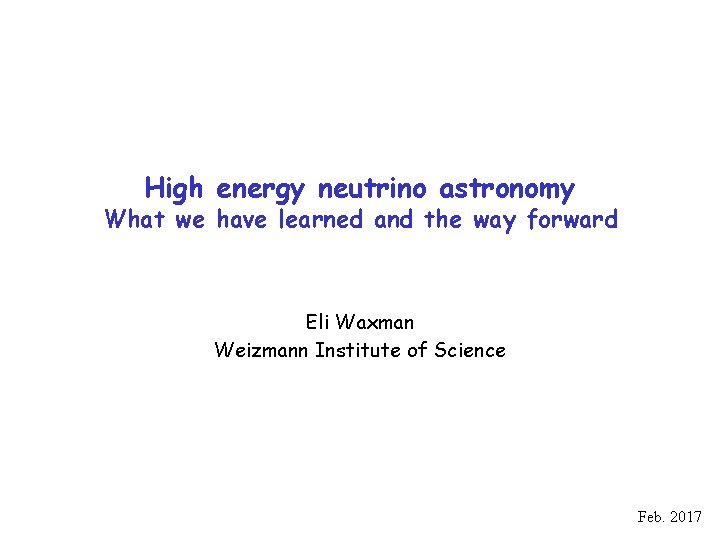
High energy neutrino astronomy What we have learned and the way forward Eli Waxman Weizmann Institute of Science Feb. 2017
![log dnd E The main driver of HE n astronomy The origin of Cosmic log [dn/d. E] The main driver of HE n astronomy: The origin of Cosmic](https://slidetodoc.com/presentation_image_h2/4f19c03c5bfdd3bd203e523f60186e16/image-2.jpg)
log [dn/d. E] The main driver of HE n astronomy: The origin of Cosmic Rays E-2. 7 E-3 Detection: Space (direct) Ground (Air-showers indirect) Open Q’s Composition: Protons Heavier (C, N, O, Fe) Lighter (Heavier? ) Composition? Confinement: Galactic G/XG transition? 1 Sources: X-Galactic 103 SN remnants(? ) 106 1010 ? ? E [Ge. V] Sources? Acceleration? [Reviews: Helder, et al. 12; Lemoine 13]
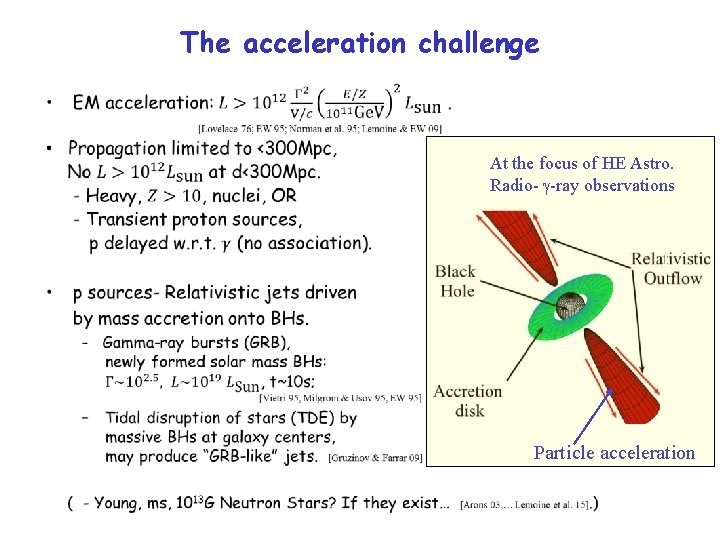
The acceleration challenge • At the focus of HE Astro. Radio- g-ray observations Particle acceleration
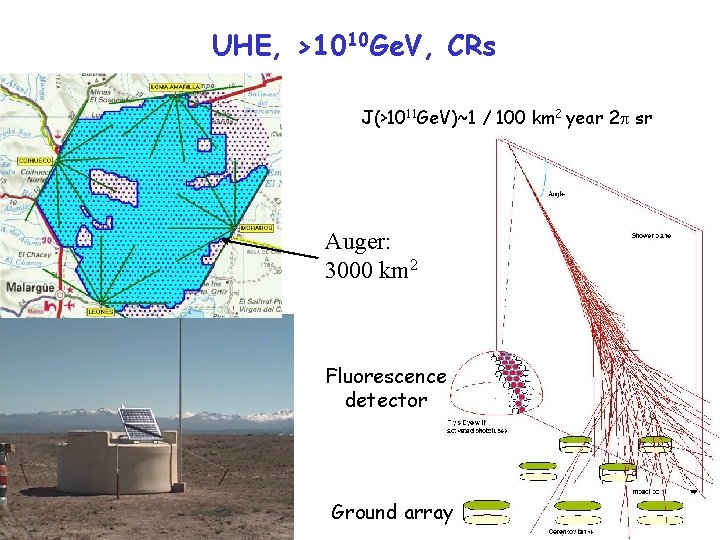
UHE, >1010 Ge. V, CRs J(>1011 Ge. V)~1 / 100 km 2 year 2 p sr 3, 000 km 2 Auger: 3000 km 2 Fluorescence detector Ground array
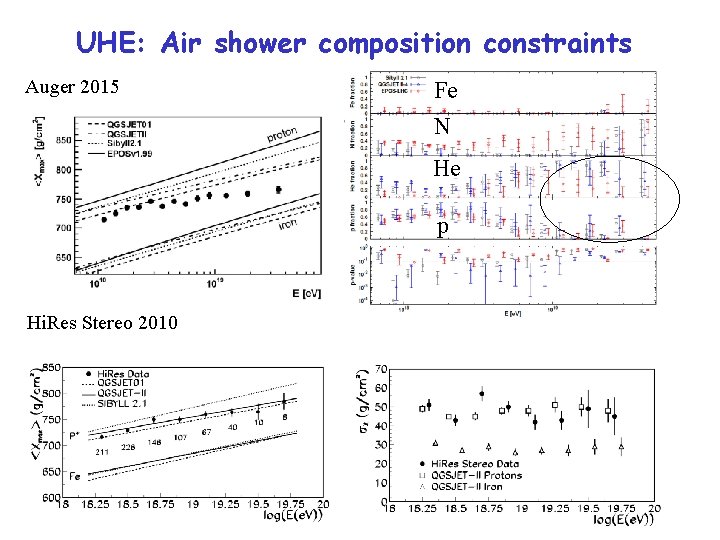
UHE: Air shower composition constraints Auger 2010 2015 Fe N He p Hi. Res Stereo 2010 Hi. Res 2005
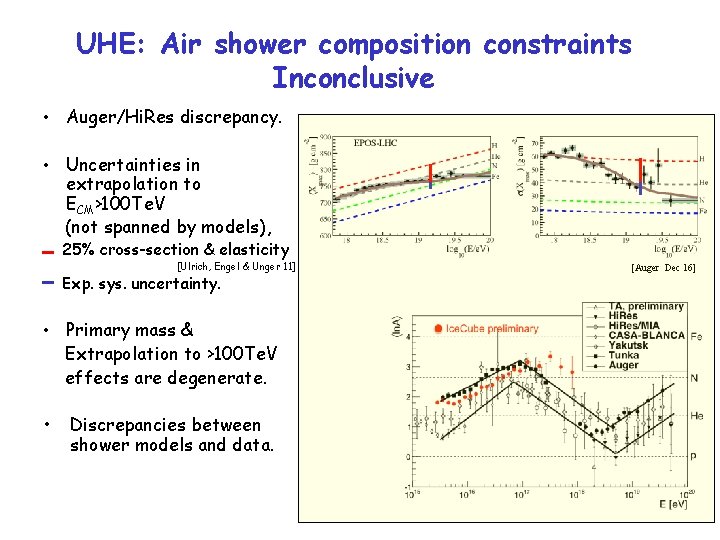
UHE: Air shower composition constraints Inconclusive • Auger/Hi. Res discrepancy. • Uncertainties in extrapolation to ECM>100 Te. V (not spanned by models), 25% cross-section & elasticity [Ulrich, Engel & Unger 11] Exp. sys. uncertainty. • Primary mass & Extrapolation to >100 Te. V effects are degenerate. • Discrepancies between shower models and data. [Auger Dec 16]
![1010 Ge V spectrum a hint to ps cteff Mpc GZK >1010 Ge. V spectrum: a hint to p’s cteff [Mpc] GZK](https://slidetodoc.com/presentation_image_h2/4f19c03c5bfdd3bd203e523f60186e16/image-7.jpg)
>1010 Ge. V spectrum: a hint to p’s cteff [Mpc] GZK
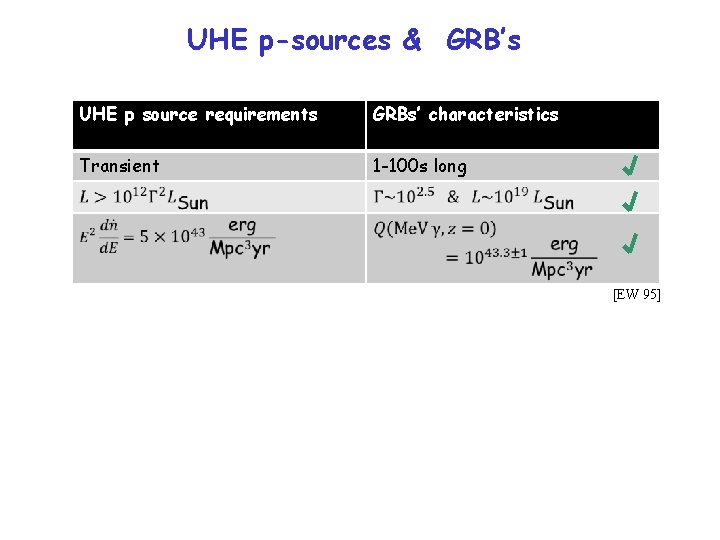
UHE p-sources & GRB’s UHE p source requirements GRBs’ characteristics Transient 1 -100 s long [EW 95]
![A mixed composition N p He Fe Auger Dec 16 A mixed composition? N p He Fe [Auger Dec 16]](https://slidetodoc.com/presentation_image_h2/4f19c03c5bfdd3bd203e523f60186e16/image-9.jpg)
A mixed composition? N p He Fe [Auger Dec 16]
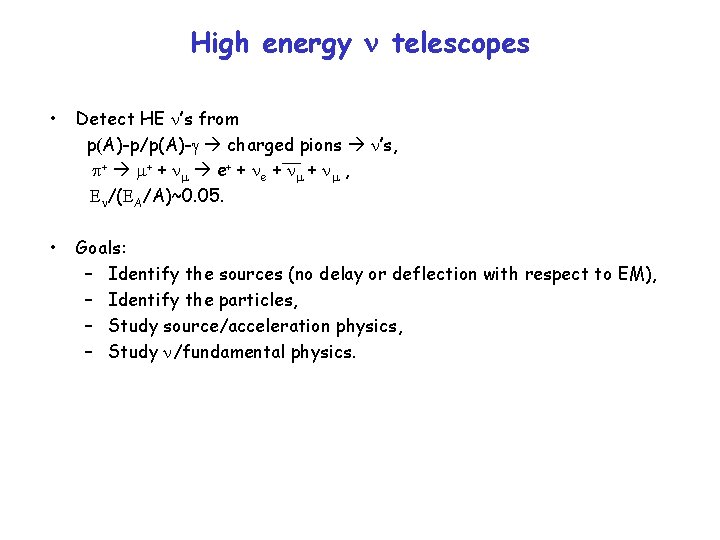
High energy n telescopes • Detect HE n’s from p(A)-p/p(A)-g charged pions n’s, p + m+ + n m e + + n e + n m , En/(EA/A)~0. 05. • Goals: – Identify the sources (no delay or deflection with respect to EM), – Identify the particles, – Study source/acceleration physics, – Study n/fundamental physics.
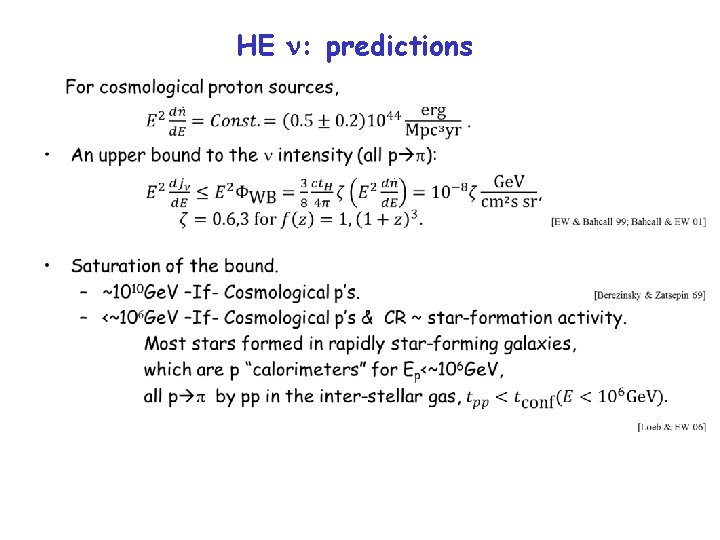
HE n: predictions •
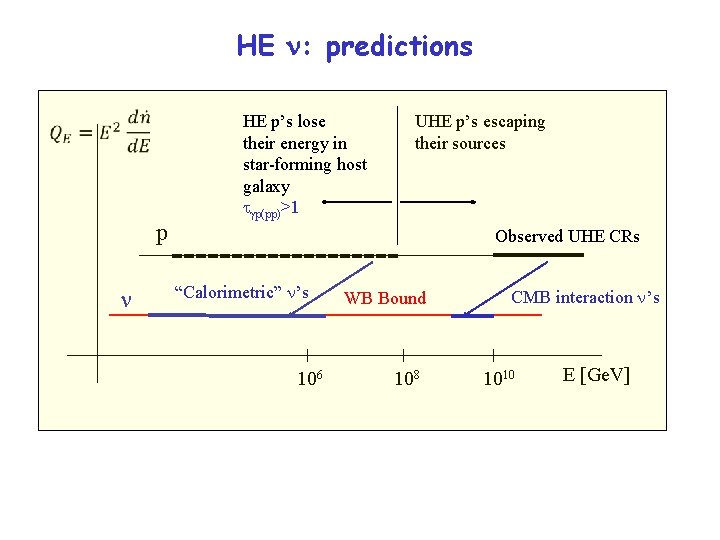
HE n: predictions p n HE p’s lose their energy in star-forming host galaxy tgp(pp)>1 UHE p’s escaping their sources Observed UHE CRs “Calorimetric” n’s 106 WB Bound 108 CMB interaction n’s 1010 E [Ge. V]
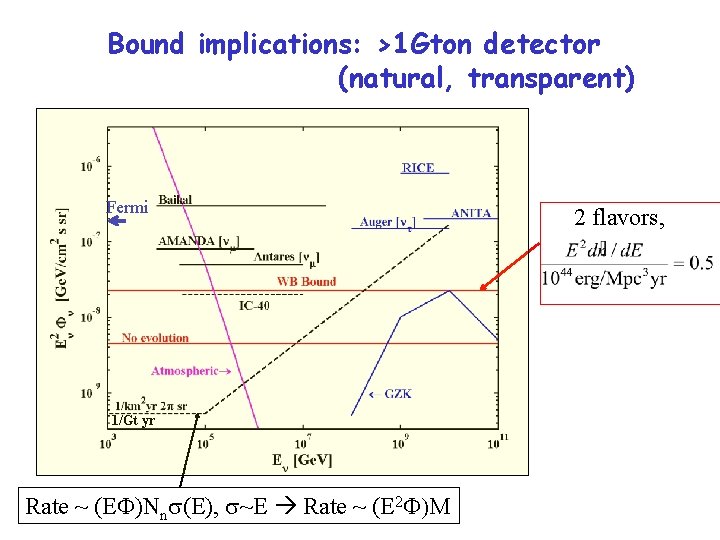
Bound implications: >1 Gton detector (natural, transparent) Fermi 1/Gt yr Rate ~ (EF)Nns(E), s~E Rate ~ (E 2 F)M 2 flavors,
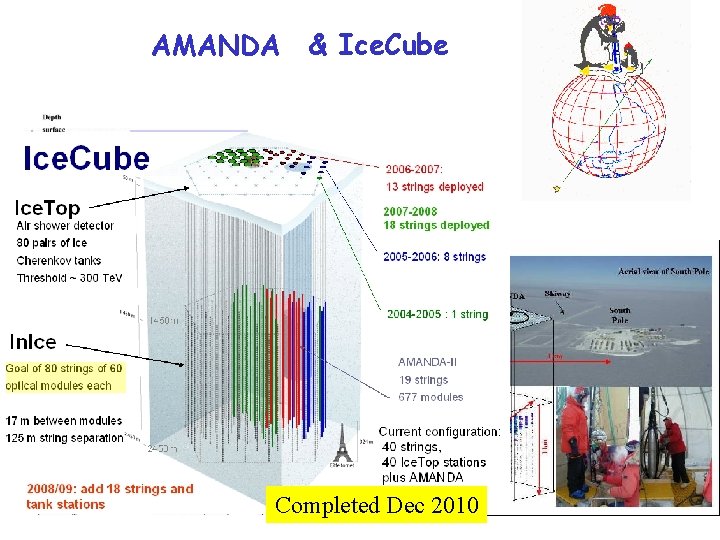
AMANDA & Ice. Cube Completed Dec 2010
![Looking up Vetoing atmospheric neutrinos Schoenert Gaisser et al 2009 Look for Events Looking up: Vetoing atmospheric neutrinos [Schoenert, Gaisser et. al 2009] • Look for: Events](https://slidetodoc.com/presentation_image_h2/4f19c03c5bfdd3bd203e523f60186e16/image-15.jpg)
Looking up: Vetoing atmospheric neutrinos [Schoenert, Gaisser et. al 2009] • Look for: Events starting within the detector, not accompanied by shower muons. • Sensitive to all flavors (for 1: 1: 1, nm induced m~20%). • Observe 4 p. • Rule out atmospheric charmed meson decay excess: Anisotropy due to downward events removal (vs isotropic astrophysical intensity). [Cartoon: N. Whitehorn]
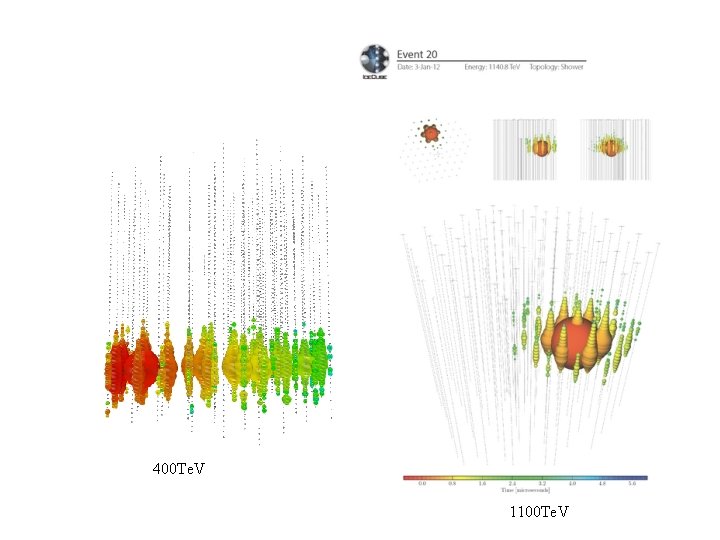
r 400 Te. V 1100 Te. V
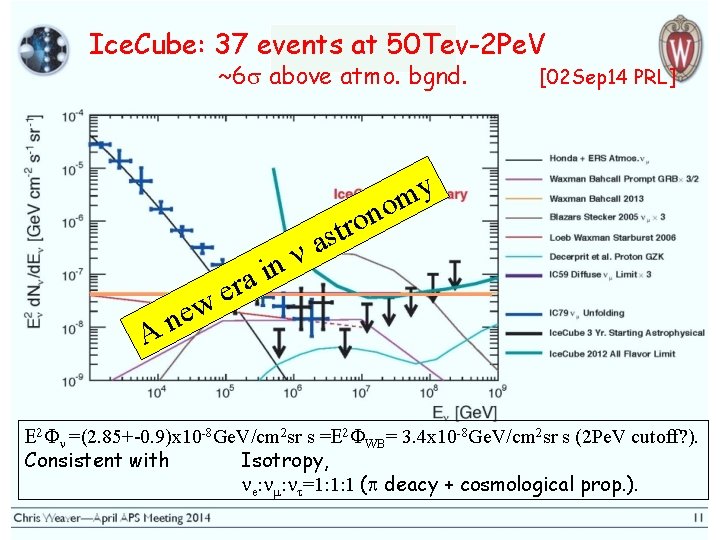
Ice. Cube: 37 events at 50 Tev-2 Pe. V ~6 s above atmo. bgnd. [02 Sep 14 PRL] y m o n stro ra e w a n in e n A E 2 Fn =(2. 85+-0. 9)x 10 -8 Ge. V/cm 2 sr s =E 2 FWB= 3. 4 x 10 -8 Ge. V/cm 2 sr s (2 Pe. V cutoff? ). Consistent with Isotropy, ne: nm: nt=1: 1: 1 (p deacy + cosmological prop. ).
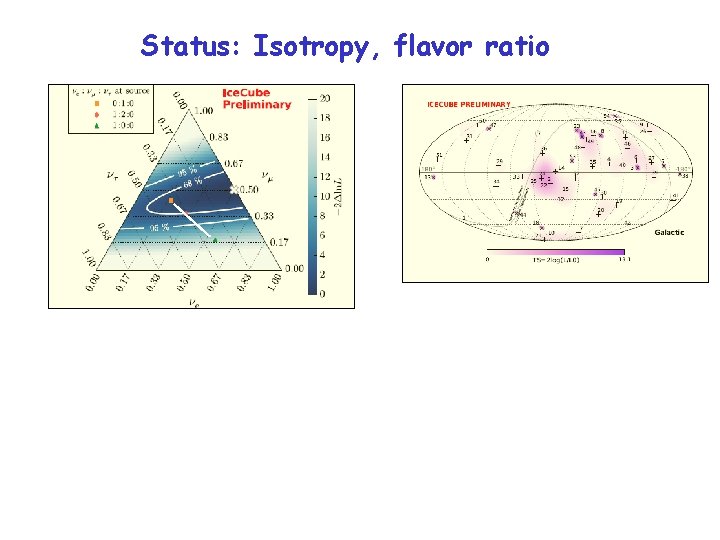
Status: Isotropy, flavor ratio
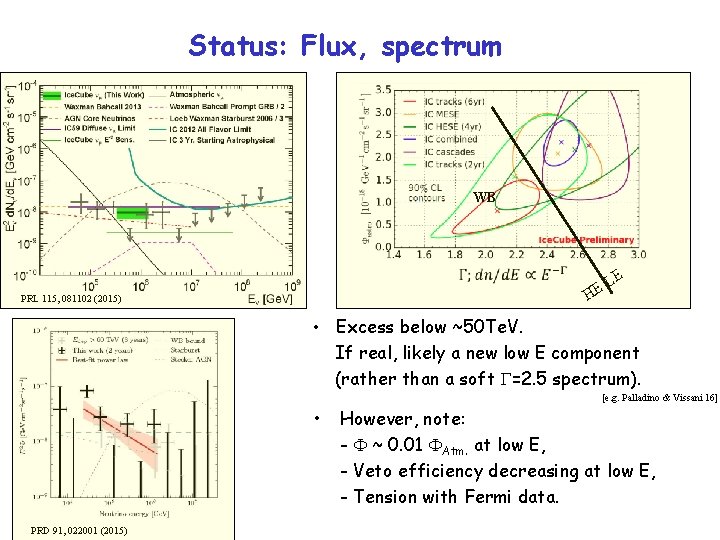
Status: Flux, spectrum WB E L E H PRL 115, 081102 (2015) • Excess below ~50 Te. V. If real, likely a new low E component (rather than a soft G=2. 5 spectrum). [e. g. Palladino & Vissani 16] • PRD 91, 022001 (2015) However, note: - F ~ 0. 01 FAtm. at low E, - Veto efficiency decreasing at low E, - Tension with Fermi data.
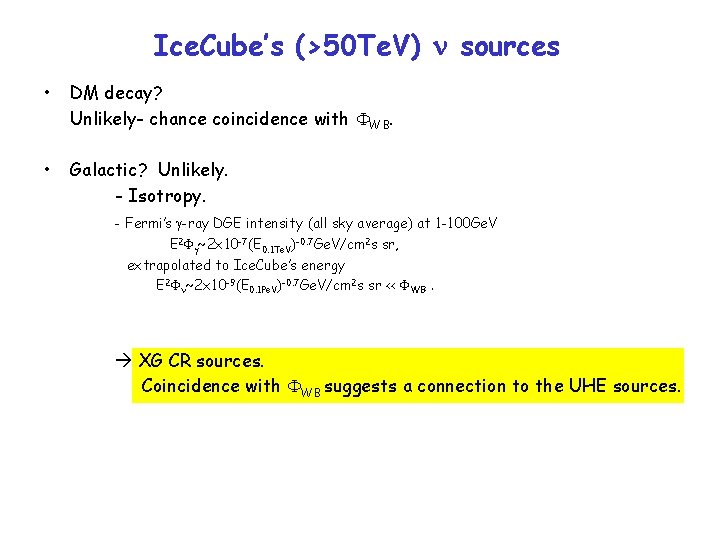
Ice. Cube’s (>50 Te. V) n sources • DM decay? Unlikely- chance coincidence with FWB. • Galactic? Unlikely. - Isotropy. - Fermi’s g-ray DGE intensity (all sky average) at 1 -100 Ge. V E 2 Fg~2 x 10 -7(E 0. 1 Te. V)-0. 7 Ge. V/cm 2 s sr, extrapolated to Ice. Cube’s energy E 2 Fn~2 x 10 -9(E 0. 1 Pe. V)-0. 7 Ge. V/cm 2 s sr << FWB. XG CR sources. Coincidence with FWB suggests a connection to the UHE sources.
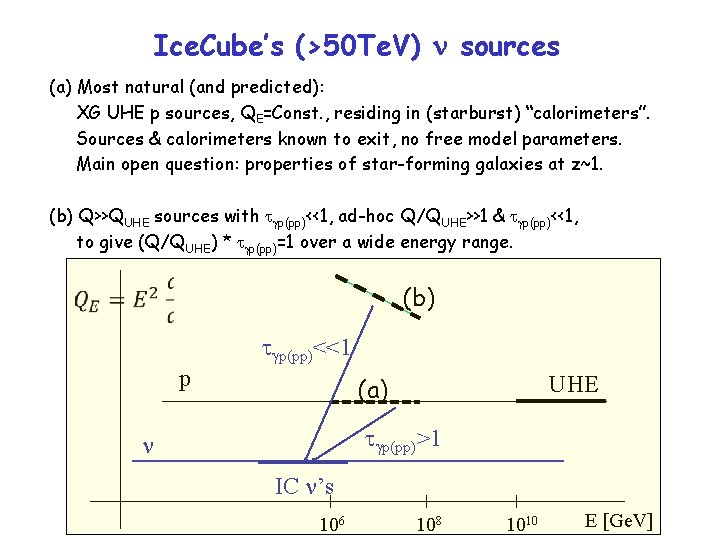
Ice. Cube’s (>50 Te. V) n sources (a) Most natural (and predicted): XG UHE p sources, QE=Const. , residing in (starburst) “calorimeters”. Sources & calorimeters known to exit, no free model parameters. Main open question: properties of star-forming galaxies at z~1. (b) Q>>QUHE sources with tgp(pp)<<1, ad-hoc Q/QUHE>>1 & tgp(pp)<<1, to give (Q/QUHE) * tgp(pp)=1 over a wide energy range. (b) tgp(pp)<<1 p UHE (a) tgp(pp)>1 n IC n’s 106 108 1010 E [Ge. V]
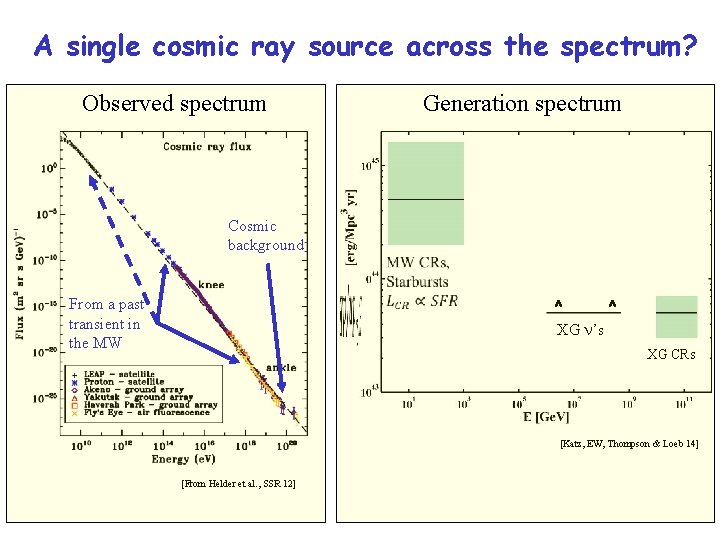
A single cosmic ray source across the spectrum? Observed spectrum Generation spectrum Cosmic background From a past transient in the MW XG n’s XG CRs [Katz, EW, Thompson & Loeb 14] [From Helder et al. , SSR 12]
![Fermis XG gray background EGB Murase EW 16 Murase 14 Fermi’s XG g-ray background [EGB] • [Murase & EW 16] Murase 14](https://slidetodoc.com/presentation_image_h2/4f19c03c5bfdd3bd203e523f60186e16/image-23.jpg)
Fermi’s XG g-ray background [EGB] • [Murase & EW 16] Murase 14
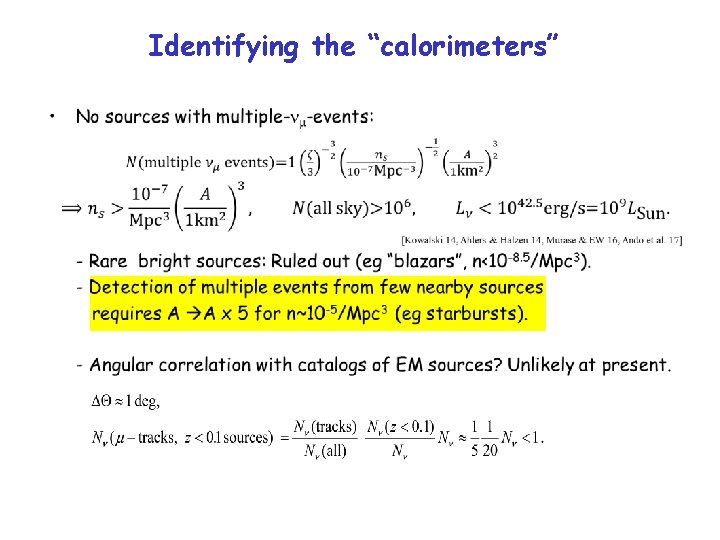
Identifying the “calorimeters” •
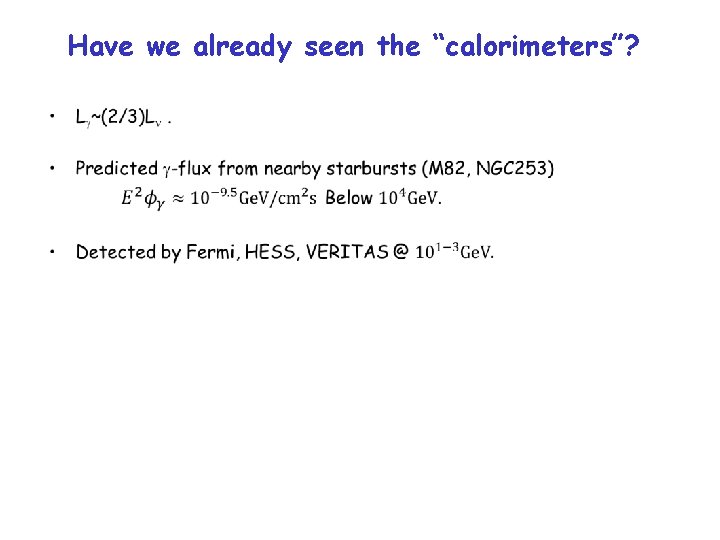
Have we already seen the “calorimeters”? •
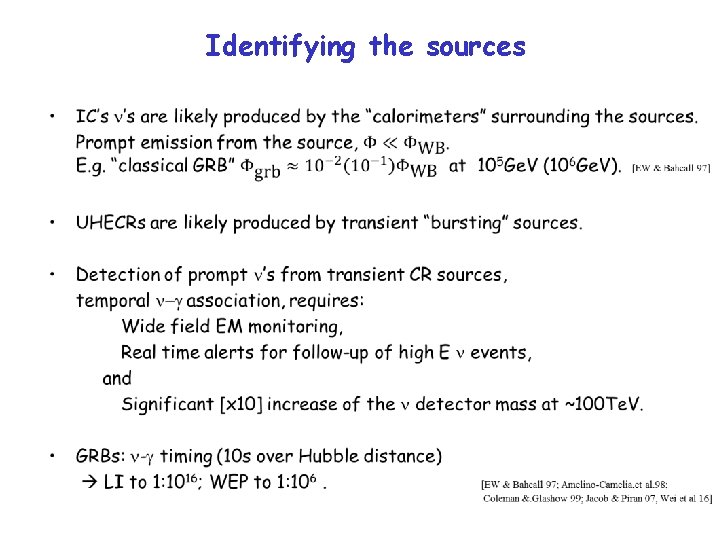
Identifying the sources •
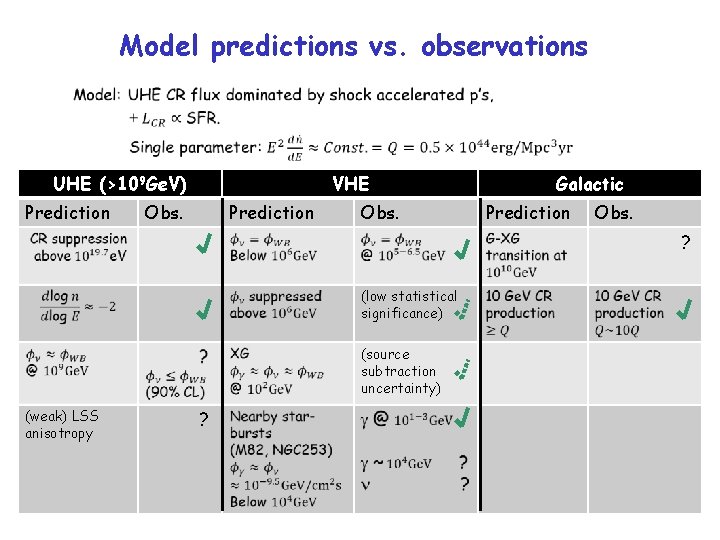
Model predictions vs. observations UHE (>109 Ge. V) Prediction VHE Obs. Prediction Obs. Galactic Prediction Obs. ? (low statistical significance) (source subtraction uncertainty) (weak) LSS anisotropy ?
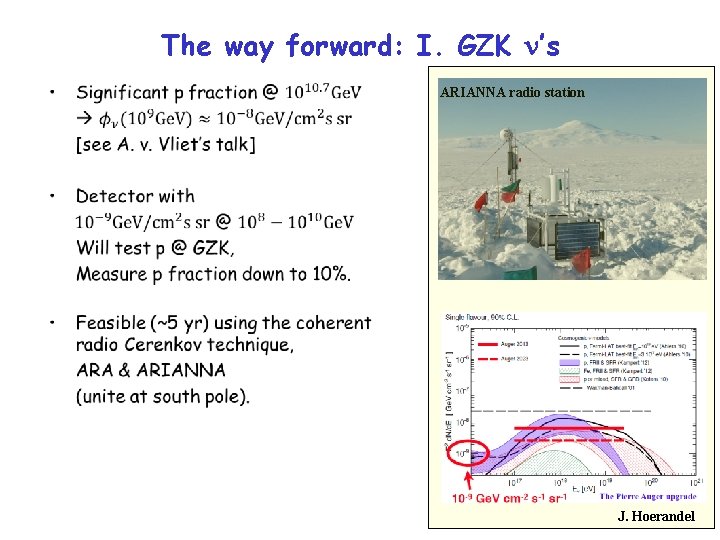
The way forward: I. GZK n’s • ARIANNA radio station J. Hoerandel
![Augers UHE limit 20136 data Auger’s UHE limit [<2013/6 data]](https://slidetodoc.com/presentation_image_h2/4f19c03c5bfdd3bd203e523f60186e16/image-29.jpg)
Auger’s UHE limit [<2013/6 data]
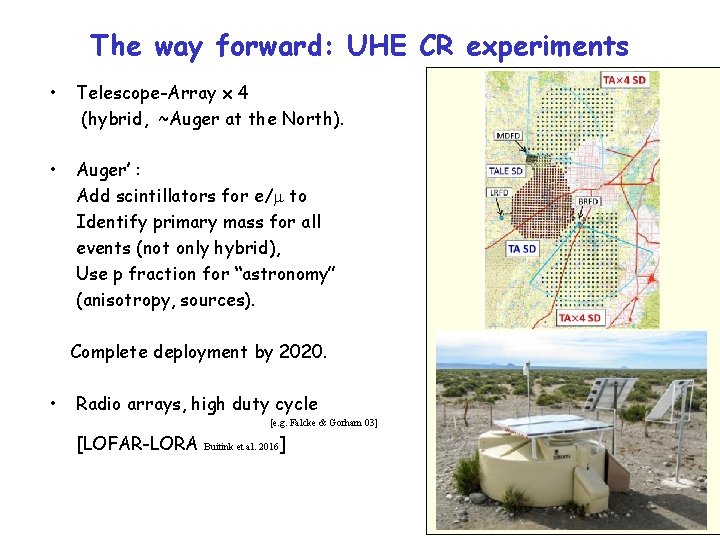
The way forward: UHE CR experiments • Telescope-Array x 4 (hybrid, ~Auger at the North). • Auger’ : Add scintillators for e/m to Identify primary mass for all events (not only hybrid), Use p fraction for “astronomy” (anisotropy, sources). Complete deployment by 2020. • Radio arrays, high duty cycle [e. g. Falcke & Gorham 03] [LOFAR-LORA Buitink et al. 2016 ]
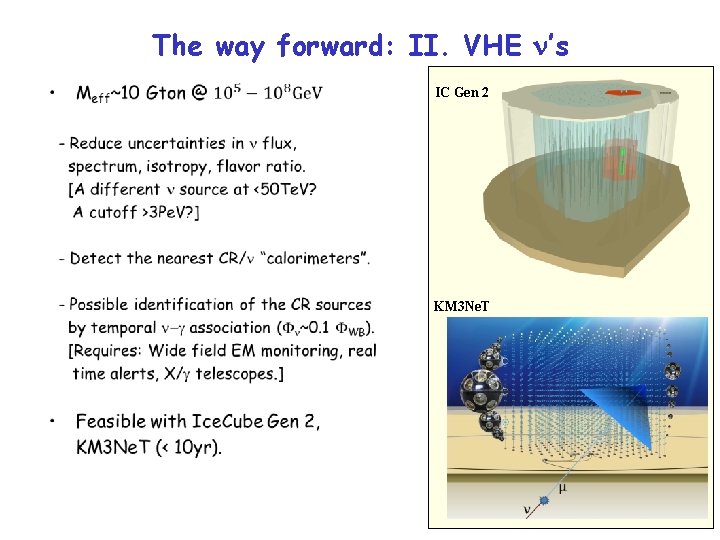
The way forward: II. VHE n’s • IC Gen 2 KM 3 Ne. T
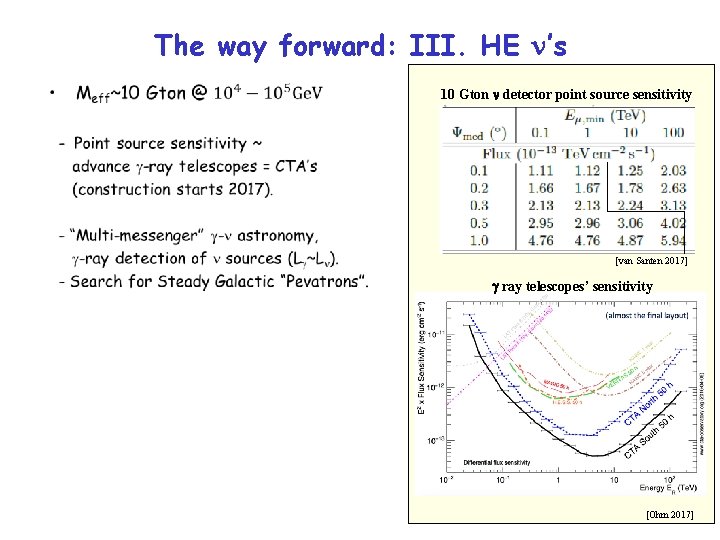
The way forward: III. HE n’s • 10 Gton n detector point source sensitivity [van Santen 2017] g ray telescopes’ sensitivity [Ohm 2017]
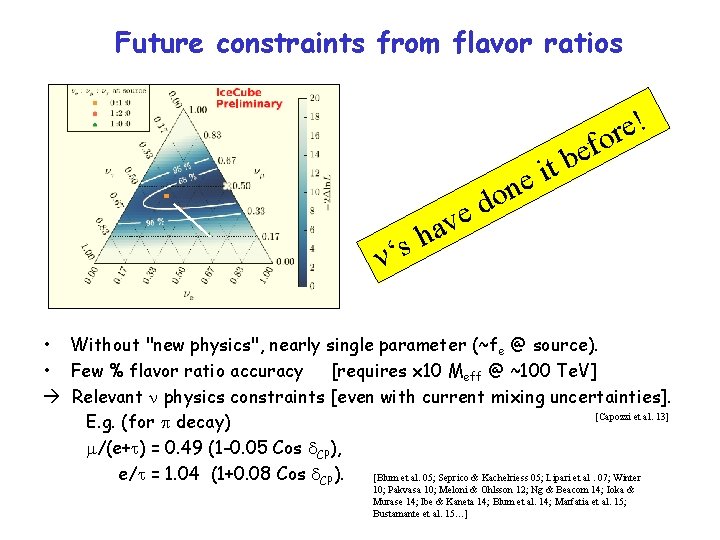
Future constraints from flavor ratios ! e r o f e tb i e on n‘s d e hav • Without "new physics", nearly single parameter (~fe @ source). • Few % flavor ratio accuracy [requires x 10 Meff @ ~100 Te. V] Relevant n physics constraints [even with current mixing uncertainties]. [Capozzi et al. 13] E. g. (for p decay) m/(e+t) = 0. 49 (1 -0. 05 Cos d. CP), e/t = 1. 04 (1+0. 08 Cos d. CP). [Blum et al. 05; Seprico & Kachelriess 05; Lipari et al. 07; Winter 10; Pakvasa 10; Meloni & Ohlsson 12; Ng & Beacom 14; Ioka & Murase 14; Ibe & Kaneta 14; Blum et al. 14; Marfatia et al. 15; Bustamante et al. 15…]
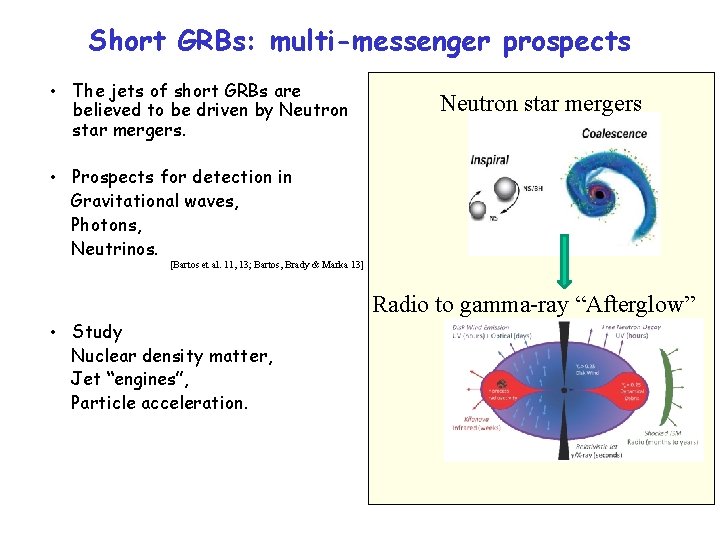
Short GRBs: multi-messenger prospects • The jets of short GRBs are believed to be driven by Neutron star mergers • Prospects for detection in Gravitational waves, Photons, Neutrinos. [Bartos et al. 11, 13; Bartos, Brady & Marka 13] Radio to gamma-ray “Afterglow” • Study Nuclear density matter, Jet “engines”, Particle acceleration.
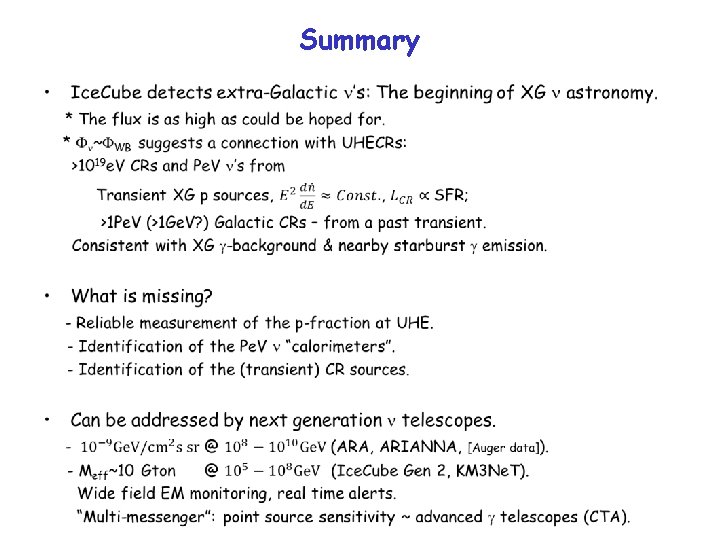
Summary •
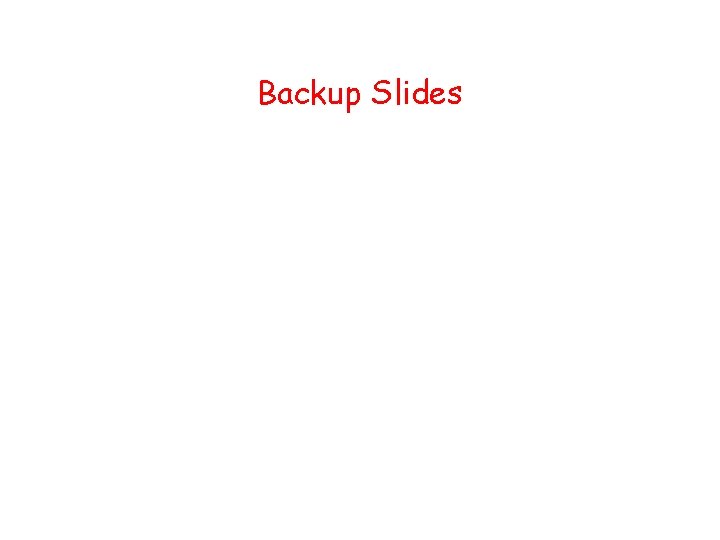
Backup Slides
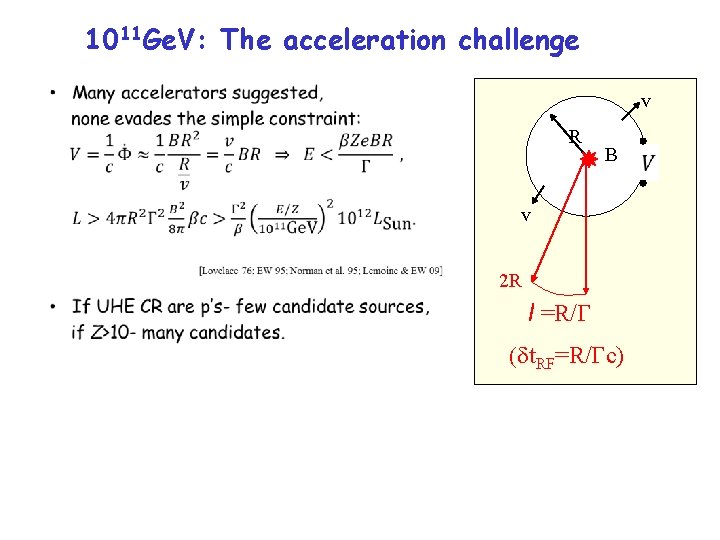
1011 Ge. V: The acceleration challenge v R B v 2 R l =R/G (dt. RF=R/Gc)
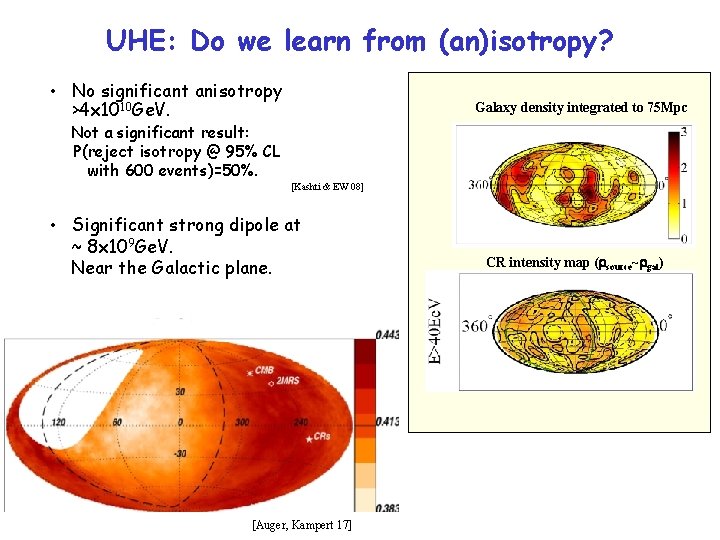
UHE: Do we learn from (an)isotropy? • No significant anisotropy >4 x 1010 Ge. V. Galaxy density integrated to 75 Mpc Not a significant result: P(reject isotropy @ 95% CL with 600 events)=50%. [Kashti & EW 08] • Significant strong dipole at ~ 8 x 109 Ge. V. Near the Galactic plane. [Auger, Kampert 17] CR intensity map (rsource~rgal)
![A note on prompt GRB ns In EW Bahcall 97 WB 10 A note on prompt GRB n’s • In [EW & Bahcall 97] WB 10%](https://slidetodoc.com/presentation_image_h2/4f19c03c5bfdd3bd203e523f60186e16/image-39.jpg)
A note on prompt GRB n’s • In [EW & Bahcall 97] WB 10% 1% GRB 0. 1 1 E (Pe. V) [Ice. Cube 16]
![Where is the GXG transition Katz EW 09 Where is the G-XG transition? [Katz & EW 09]](https://slidetodoc.com/presentation_image_h2/4f19c03c5bfdd3bd203e523f60186e16/image-40.jpg)
Where is the G-XG transition? [Katz & EW 09]
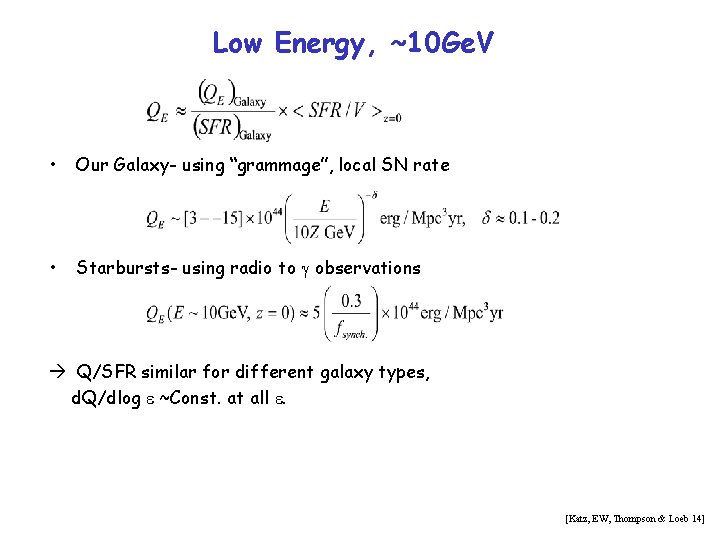
Low Energy, ~10 Ge. V • Our Galaxy- using “grammage”, local SN rate • Starbursts- using radio to g observations Q/SFR similar for different galaxy types, d. Q/dlog e ~Const. at all e. [Katz, EW, Thompson & Loeb 14]
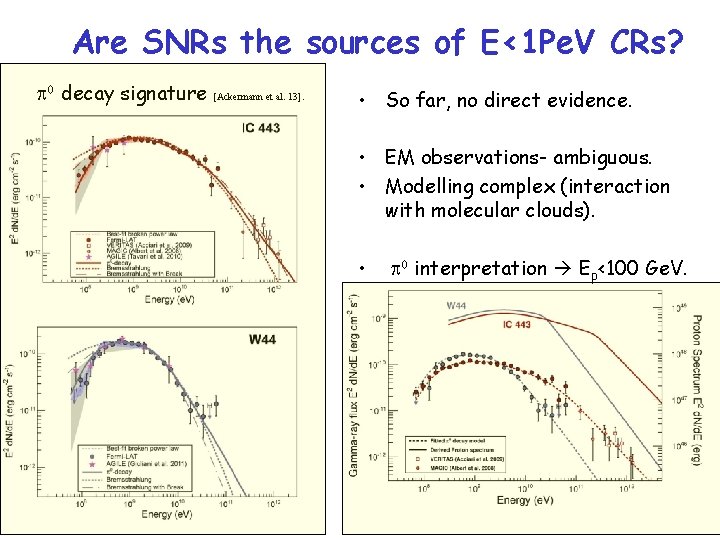
Are SNRs the sources of E<1 Pe. V CRs? p 0 decay signature [Ackermann et al. 13]. • So far, no direct evidence. • EM observations- ambiguous. • Modelling complex (interaction with molecular clouds). • p 0 interpretation Ep<100 Ge. V.
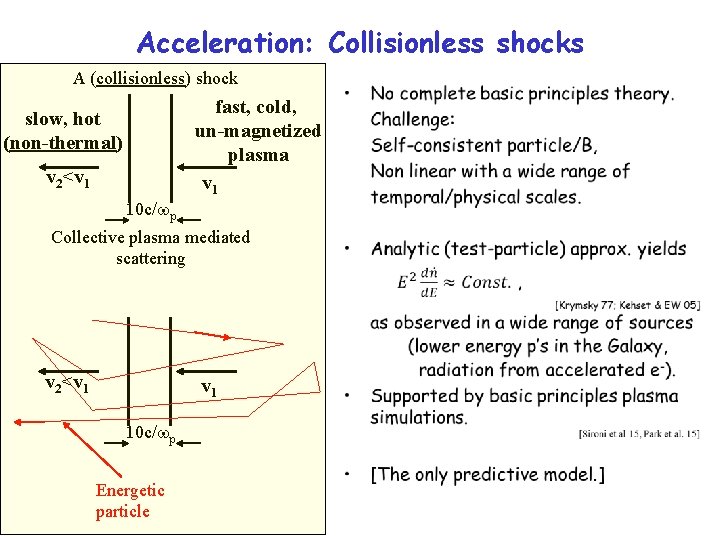
Acceleration: Collisionless shocks A (collisionless) shock fast, cold, un-magnetized plasma v 1 slow, hot (non-thermal) v 2<v 1 10 c/wp Collective plasma mediated scattering v 2<v 1 10 c/wp Energetic particle
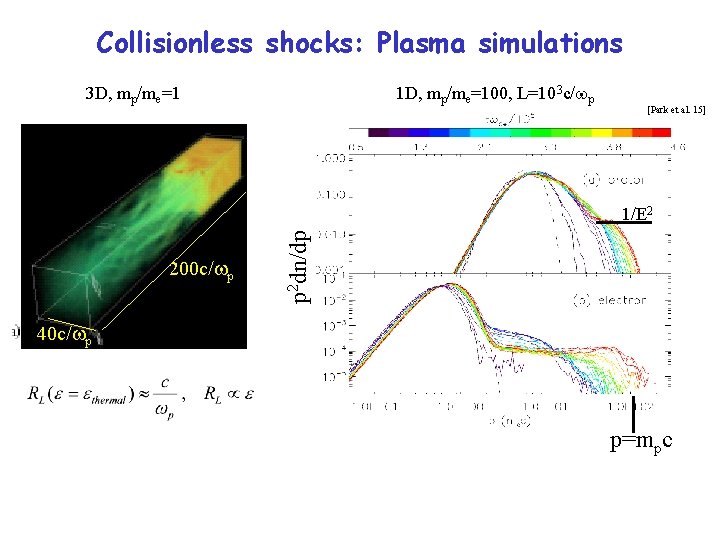
Collisionless shocks: Plasma simulations 3 D, mp/me=1 1 D, mp/me=100, L=103 c/wp [Park et al. 15] 200 c/wp p 2 dn/dp 1/E 2 40 c/wp p=mpc
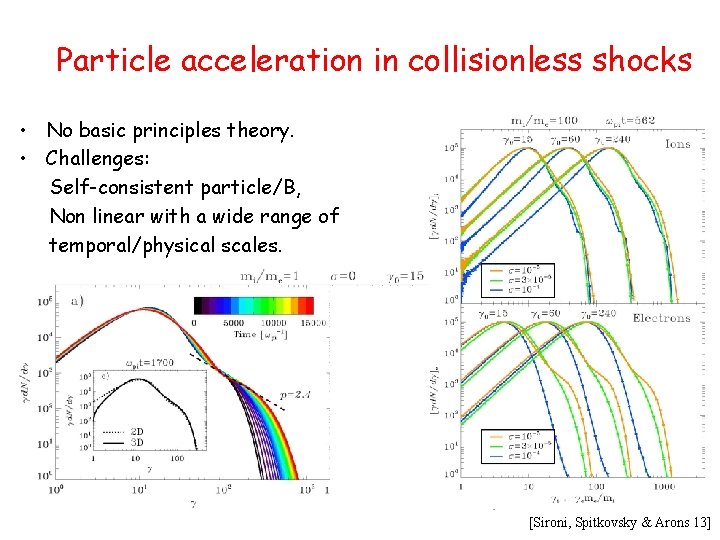
Particle acceleration in collisionless shocks • No basic principles theory. • Challenges: Self-consistent particle/B, Non linear with a wide range of temporal/physical scales. [Sironi, Spitkovsky & Arons 13]
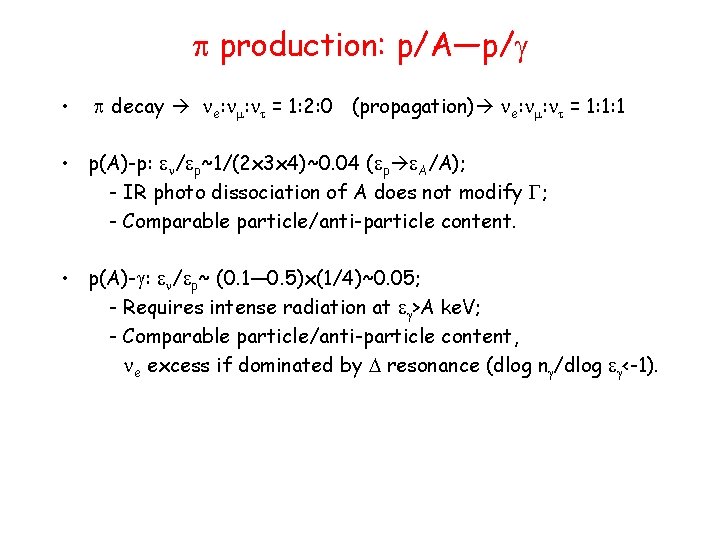
p production: p/A—p/g • p decay ne: nm: nt = 1: 2: 0 (propagation) ne: nm: nt = 1: 1: 1 • p(A)-p: en/ep~1/(2 x 3 x 4)~0. 04 (ep e. A/A); - IR photo dissociation of A does not modify G; - Comparable particle/anti-particle content. • p(A)-g: en/ep~ (0. 1— 0. 5)x(1/4)~0. 05; - Requires intense radiation at eg>A ke. V; - Comparable particle/anti-particle content, ne excess if dominated by D resonance (dlog ng/dlog eg<-1).
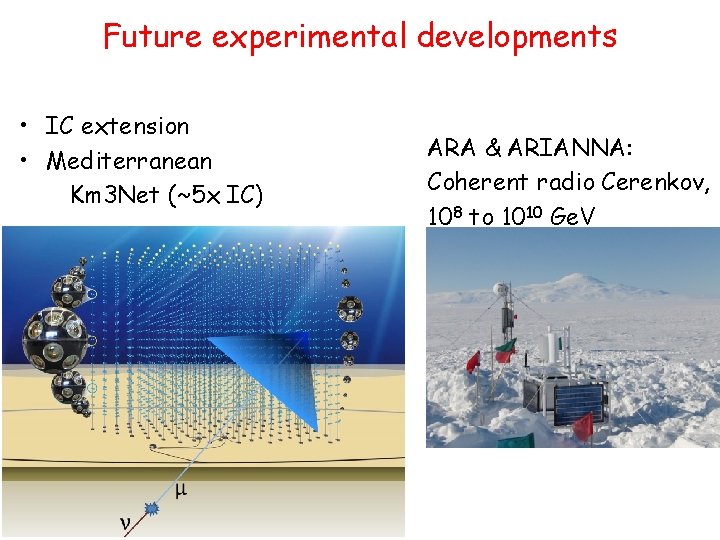
Future experimental developments • IC extension • Mediterranean Km 3 Net (~5 x IC) • ARA & ARIANNA: Coherent radio Cerenkov, 108 to 1010 Ge. V
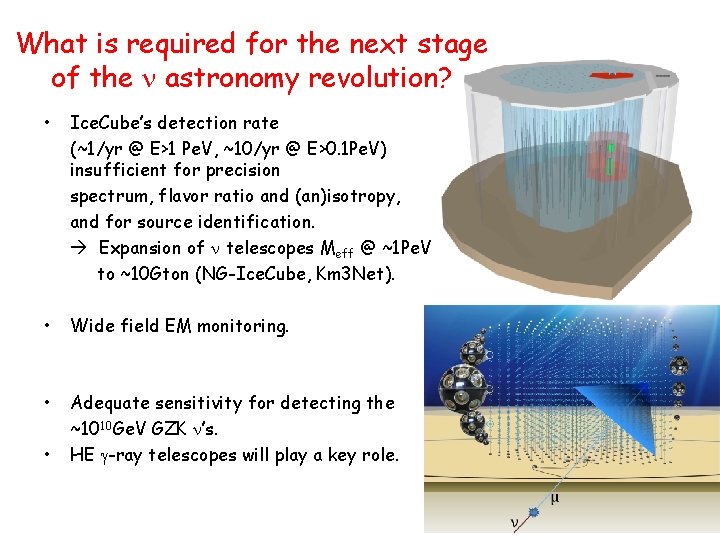
What is required for the next stage of the n astronomy revolution? • Ice. Cube’s detection rate (~1/yr @ E>1 Pe. V, ~10/yr @ E>0. 1 Pe. V) insufficient for precision spectrum, flavor ratio and (an)isotropy, and for source identification. Expansion of n telescopes Meff @ ~1 Pe. V to ~10 Gton (NG-Ice. Cube, Km 3 Net). • Wide field EM monitoring. • Adequate sensitivity for detecting the ~1010 Ge. V GZK n’s. HE g-ray telescopes will play a key role. •
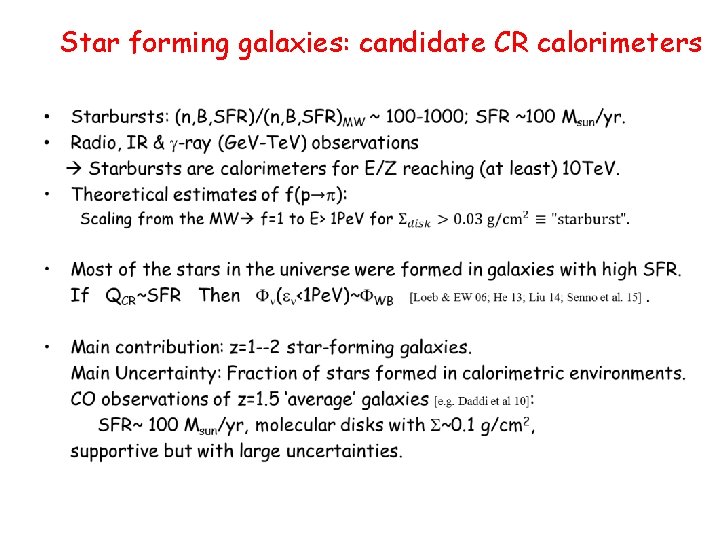
Star forming galaxies: candidate CR calorimeters •
![Rev Sci Inst [Rev. Sci. Inst. ]](https://slidetodoc.com/presentation_image_h2/4f19c03c5bfdd3bd203e523f60186e16/image-50.jpg)
[Rev. Sci. Inst. ]
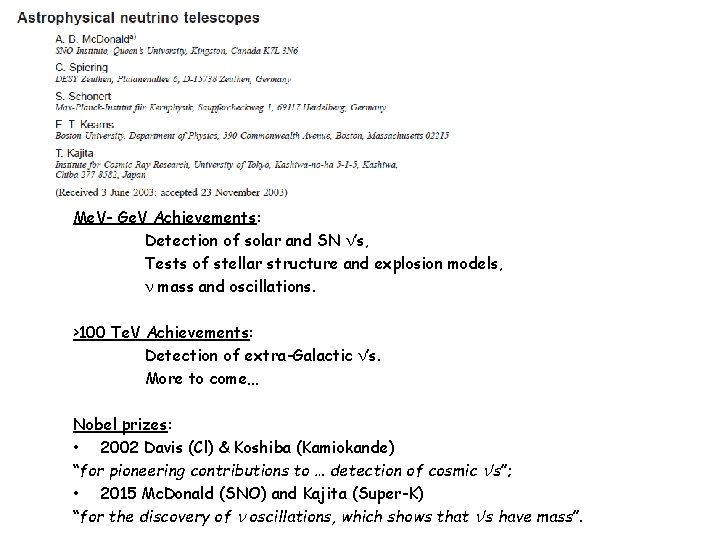
Me. V- Ge. V Achievements: Detection of solar and SN n’s, Tests of stellar structure and explosion models, n mass and oscillations. >100 Te. V Achievements: Detection of extra-Galactic n’s. More to come… Nobel prizes: • 2002 Davis (Cl) & Koshiba (Kamiokande) “for pioneering contributions to … detection of cosmic n’s”; • 2015 Mc. Donald (SNO) and Kajita (Super-K) “for the discovery of n oscillations, which shows that n’s have mass”.
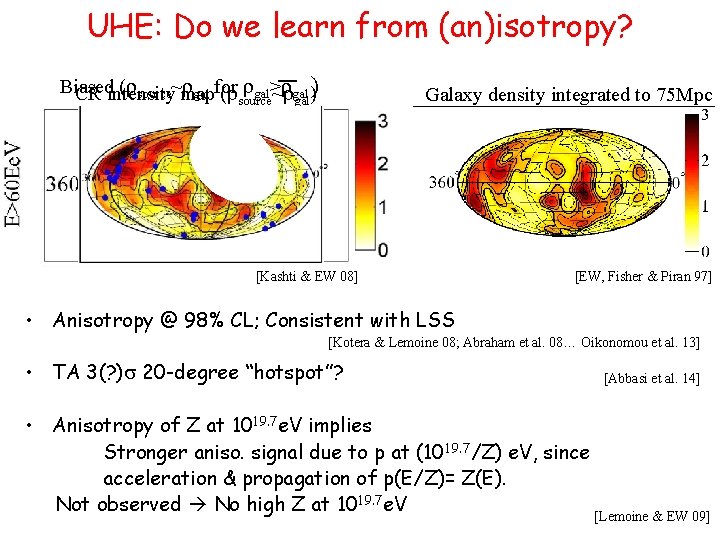
UHE: Do we learn from (an)isotropy? Biased (rsource~map rgal for rgal>~rrgal )) CR intensity (rsource gal Galaxy density integrated to 75 Mpc [Kashti & EW 08] [EW, Fisher & Piran 97] • Anisotropy @ 98% CL; Consistent with LSS [Kotera & Lemoine 08; Abraham et al. 08… Oikonomou et al. 13] • TA 3(? )s 20 -degree “hotspot”? [Abbasi et al. 14] • Anisotropy of Z at 1019. 7 e. V implies Stronger aniso. signal due to p at (10 19. 7/Z) e. V, since acceleration & propagation of p(E/Z)= Z(E). Not observed No high Z at 1019. 7 e. V [Lemoine & EW 09]
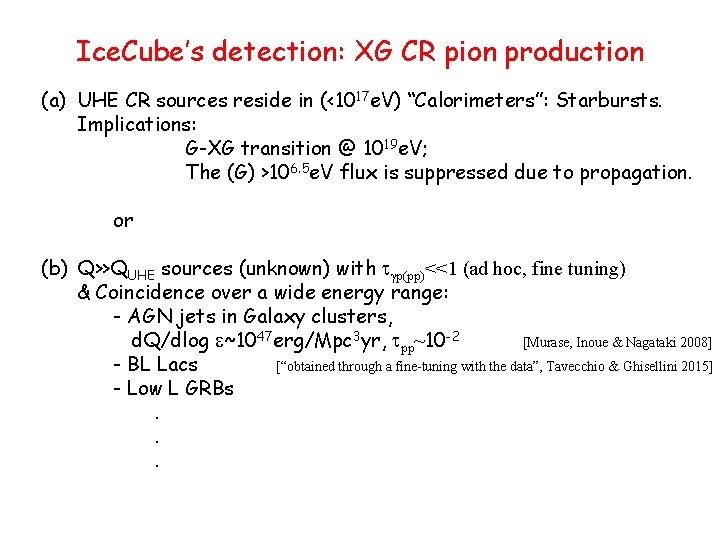
Ice. Cube’s detection: XG CR pion production (a) UHE CR sources reside in (<1017 e. V) “Calorimeters”: Starbursts. Implications: G-XG transition @ 1019 e. V; The (G) >106. 5 e. V flux is suppressed due to propagation. or (b) Q>>QUHE sources (unknown) with tgp(pp)<<1 (ad hoc, fine tuning) & Coincidence over a wide energy range: - AGN jets in Galaxy clusters, d. Q/dlog e~1047 erg/Mpc 3 yr, tpp~10 -2 [Murase, Inoue & Nagataki 2008] - BL Lacs [“obtained through a fine-tuning with the data”, Tavecchio & Ghisellini 2015] - Low L GRBs. . .
Learning astronomy by doing astronomy
Learning astronomy by doing astronomy answers
Learning astronomy by doing astronomy activity 1 answers
(-5)-(-12)
Dotterkärna
Leptons
Emission spectrum of sodium
Neutrino
Neutrino
Lekka cząstka elementarna mion elektron lub neutrino
Who discovered neutrino
Neutrino
Neutrino
Neutrino density
Neutrino
Neutrino load balancer
Neutrino
Neutrino symbol
Neutrino
Neutrino
Solar neutrino
Neutrino
Solar neutrino
Nakamura
Neutrino mass
Neutrino
Neutrino interaction with matter
Neutrino mass
6 the periodic table
Do metals have high ionization energy
Once upon a time gabriel okara
What was our previous lesson
Learn from the ants
I have learned that cause and effect
You must unlearn what you have learned
Aprender future tense
What have you learnt from the story
Example of concupiscence in ethics
In the previous lesson
In your previous lesson you have learned
While their left hands search my empty pockets
In the previous lesson you have learned
What is something you have learned lately
Lessons from life of pi
I have already learned
Now that you have learned about this concept
Have we learned from the past
3 most important things in life essay
A 3d shape with 6 faces 8 vertices and 12 edges
Energy energy transfer and general energy analysis
Energy energy transfer and general energy analysis
What is astronomy
Claudius ptolemy astronomy contributions
Astronomy greek roots