GEOLOGICAL AND GEOCHEMICAL EXPLORATION Dr Ahmed Ali Madani
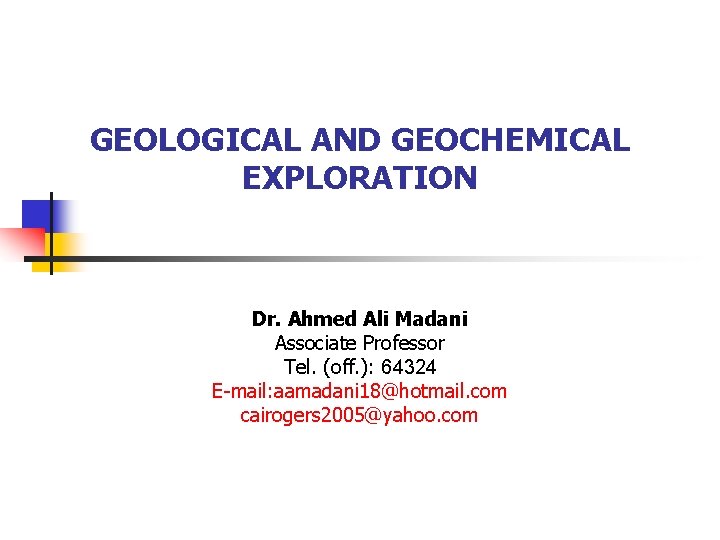
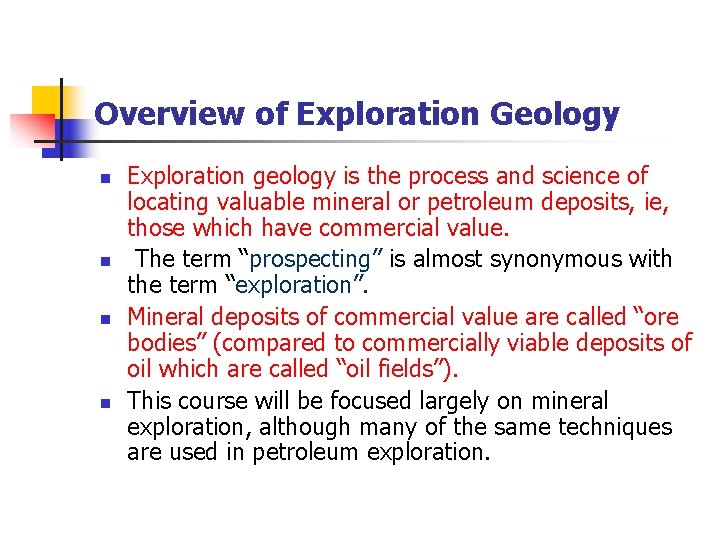
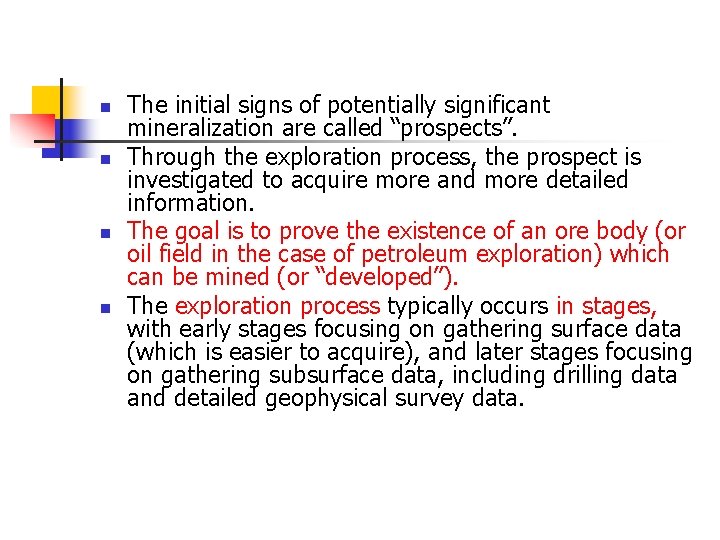
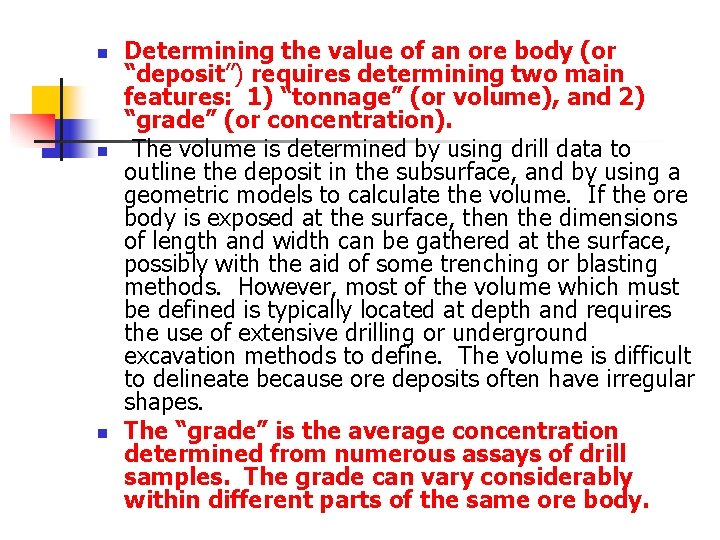
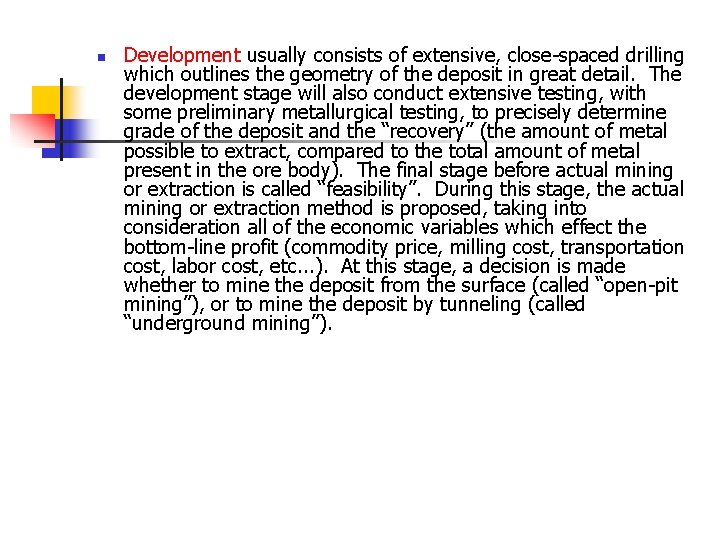
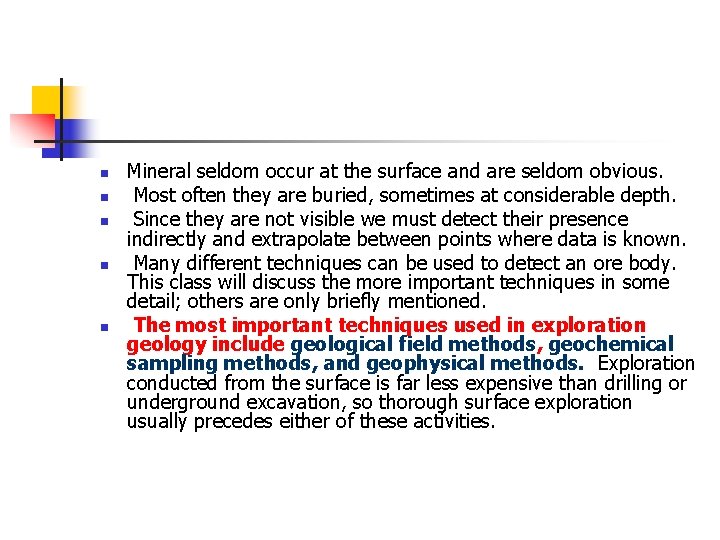
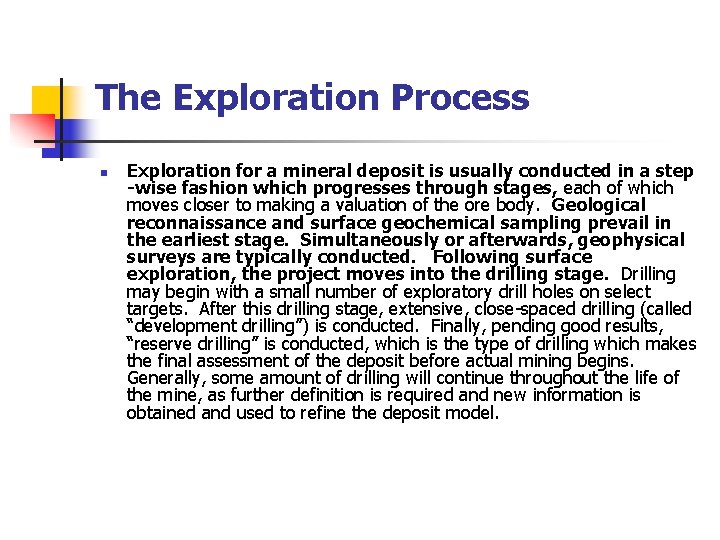
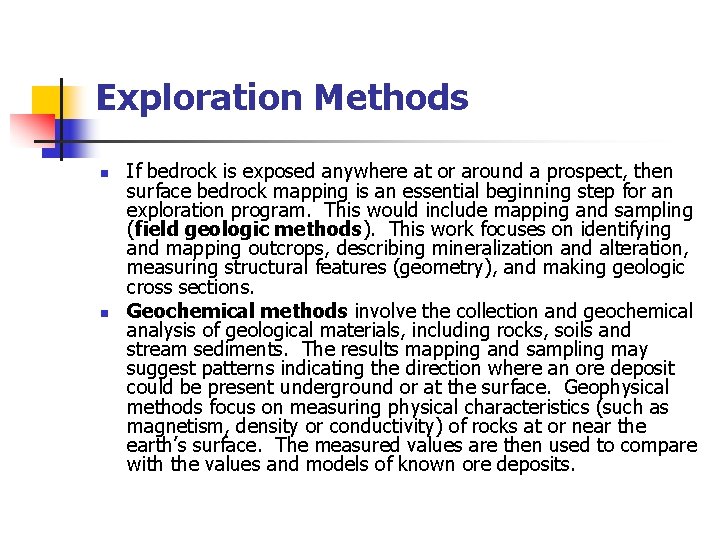
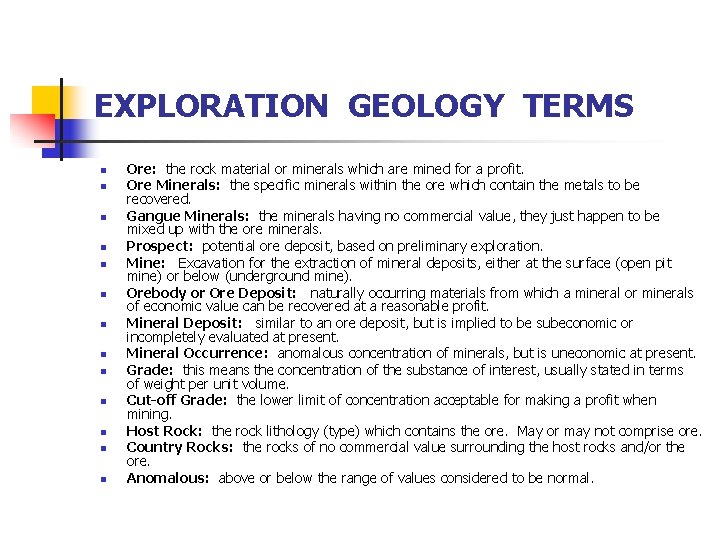
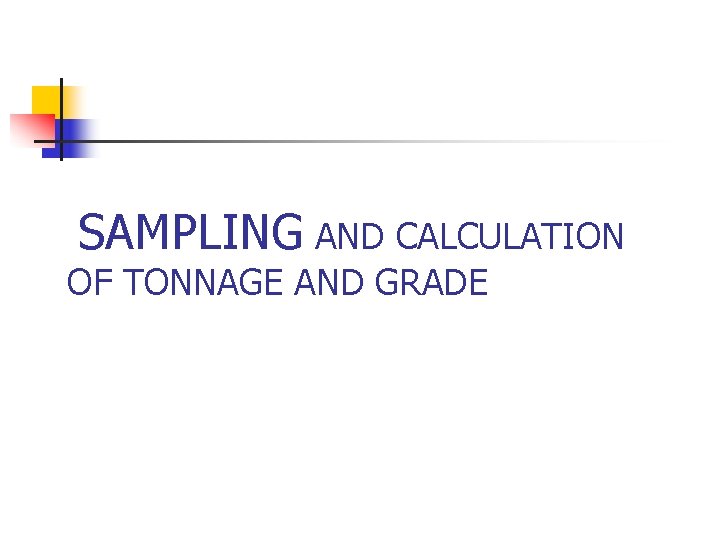
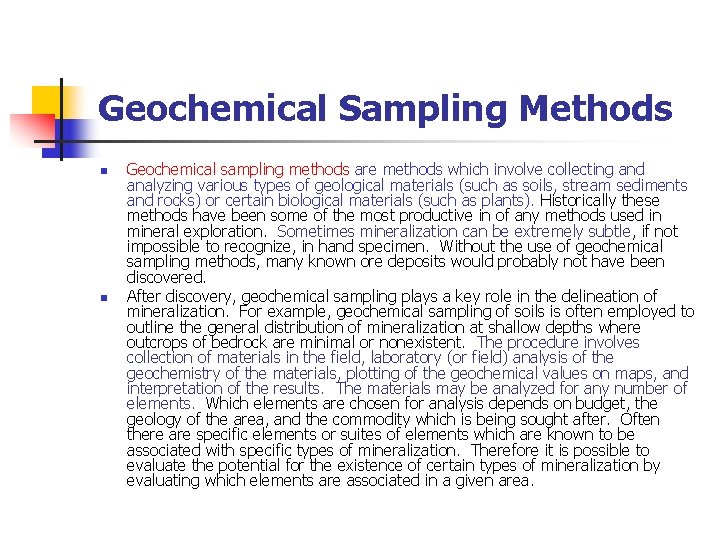
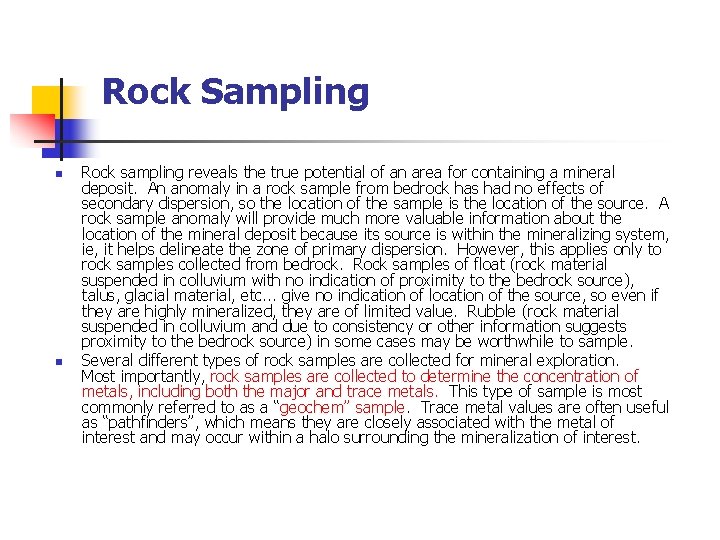
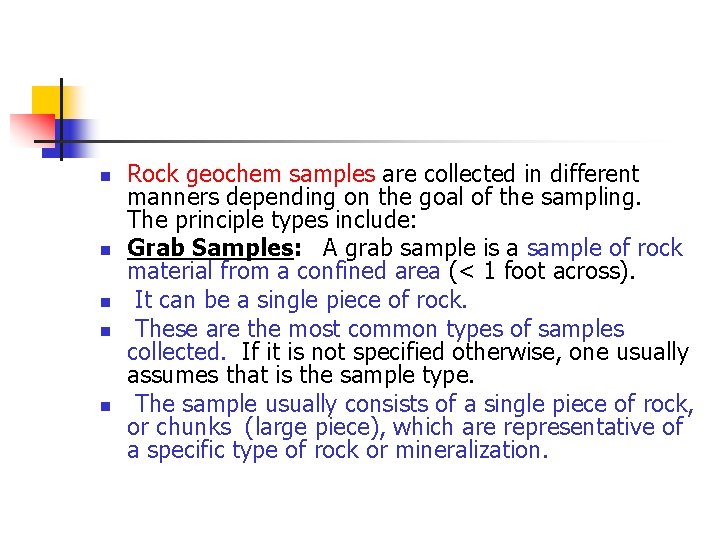
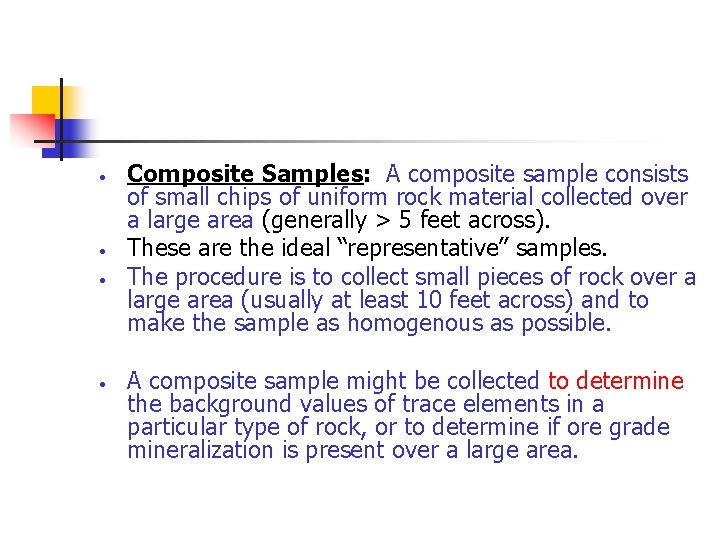
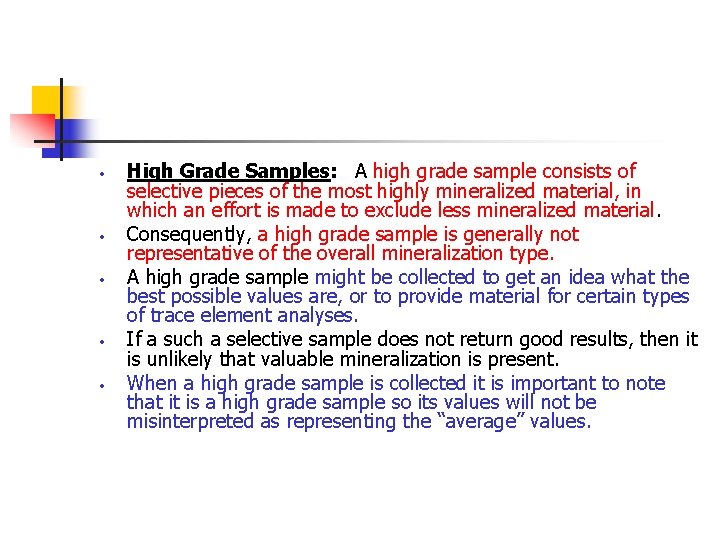
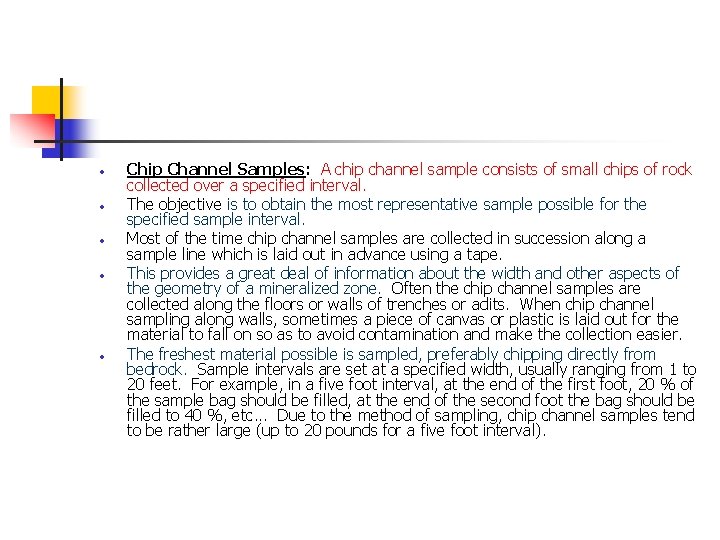
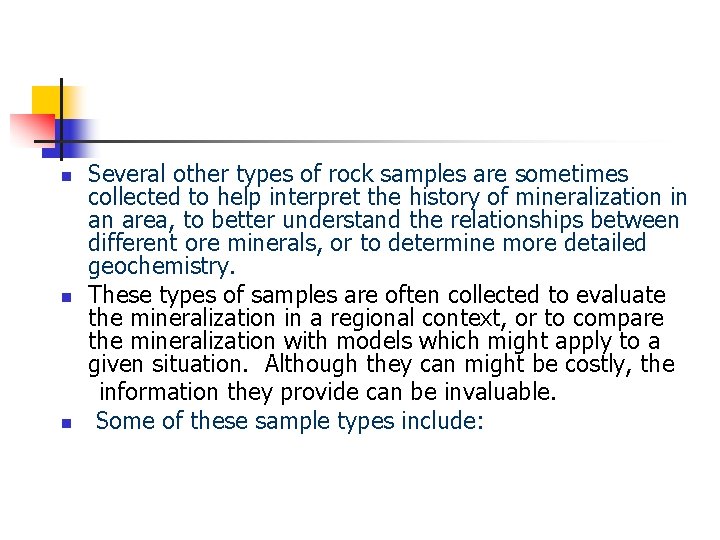
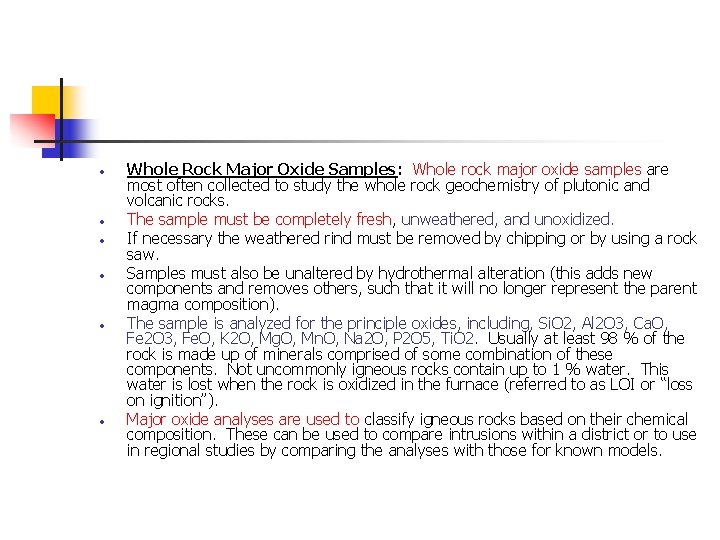
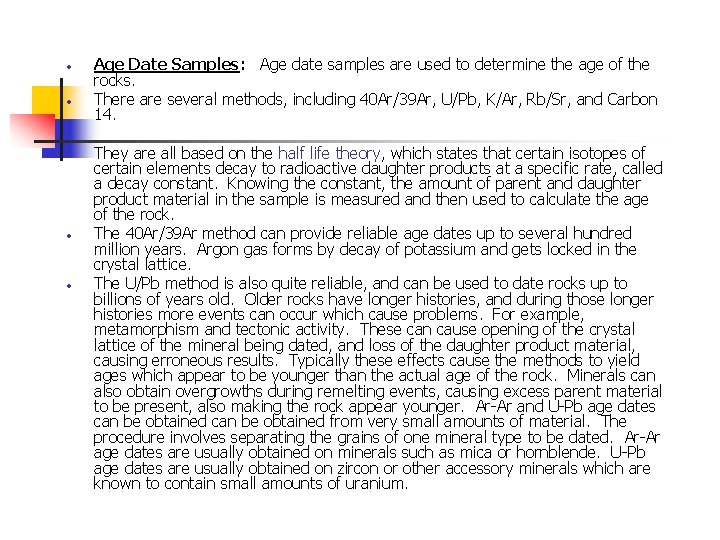
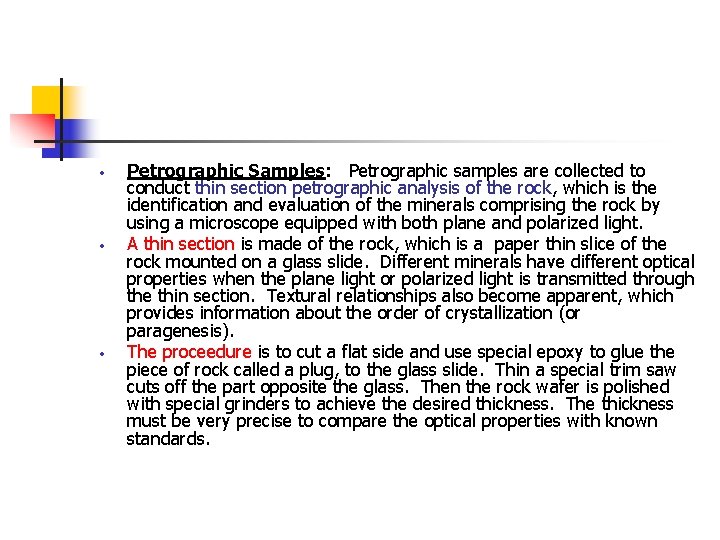
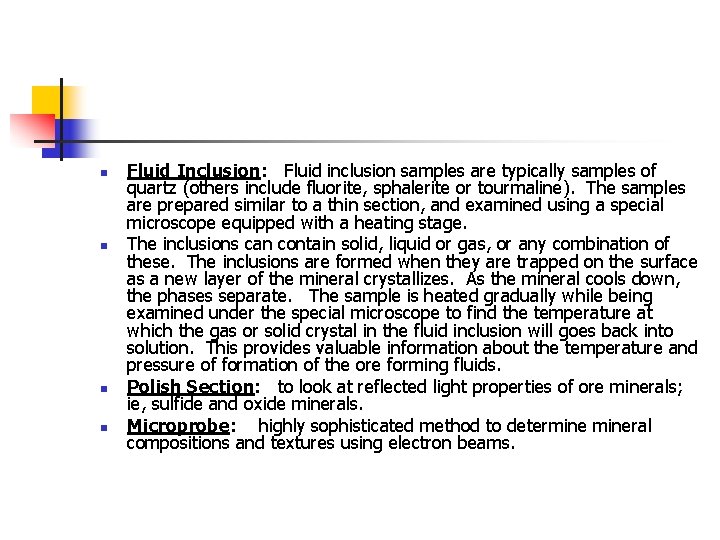
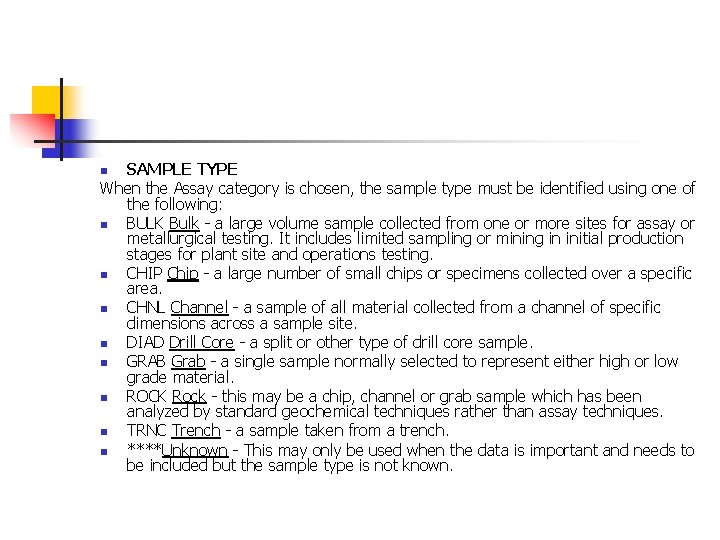
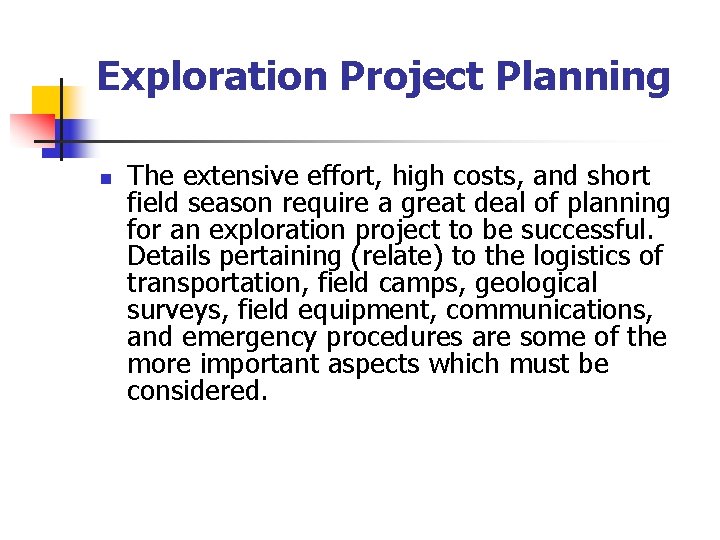
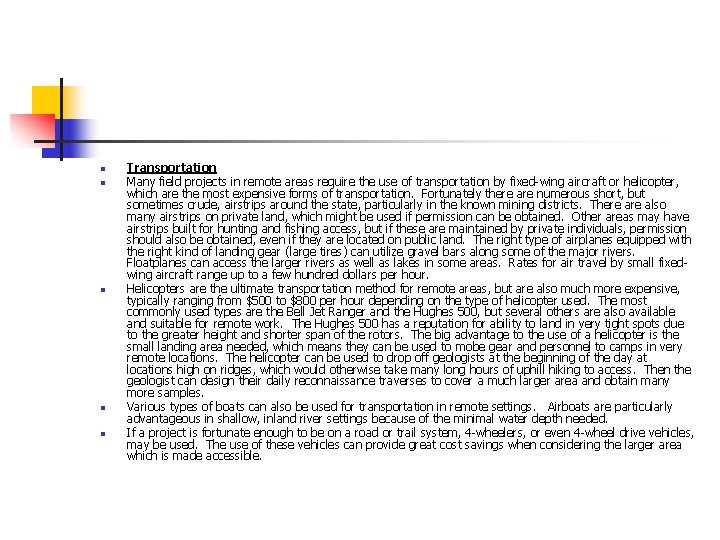
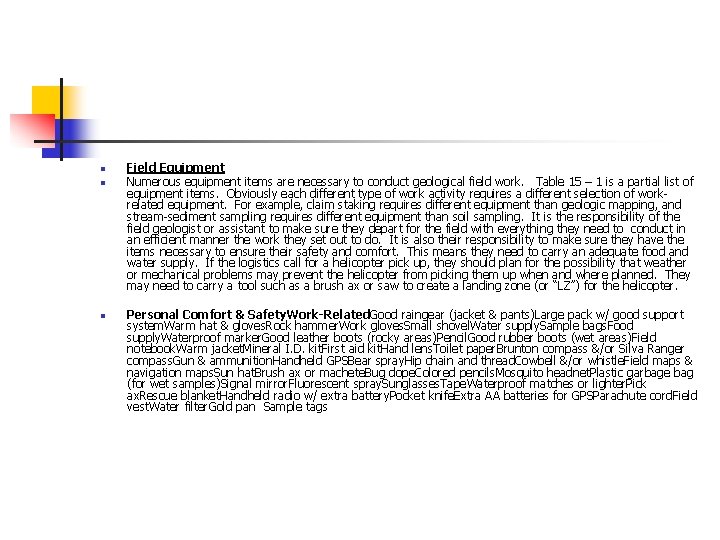
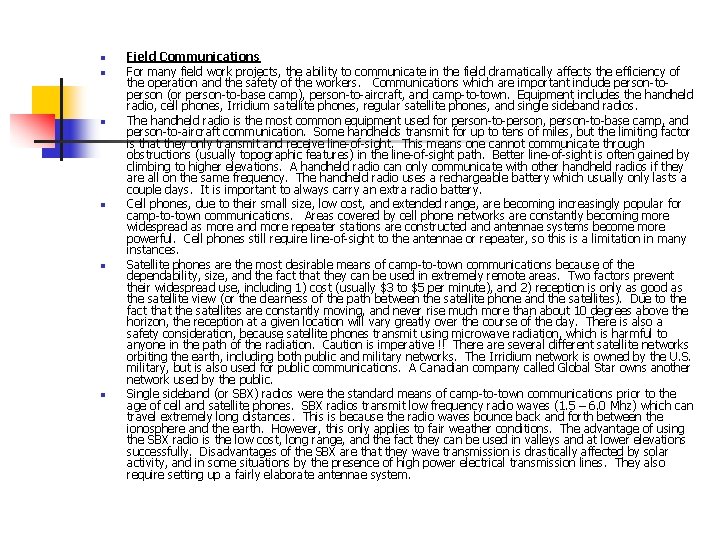
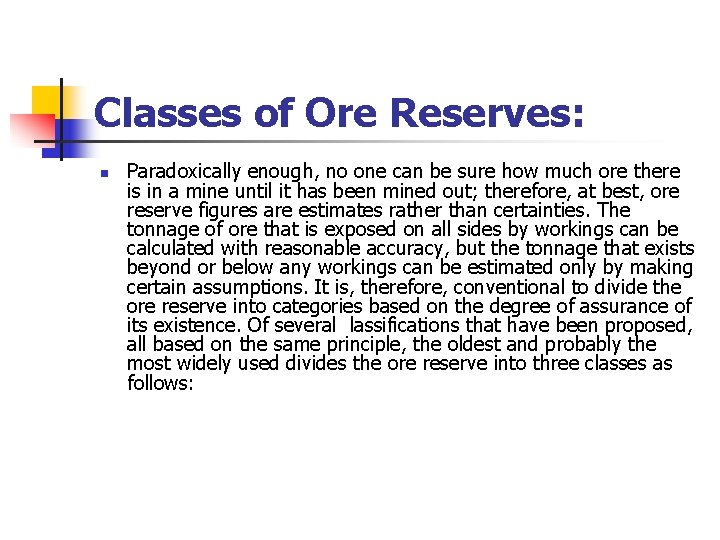
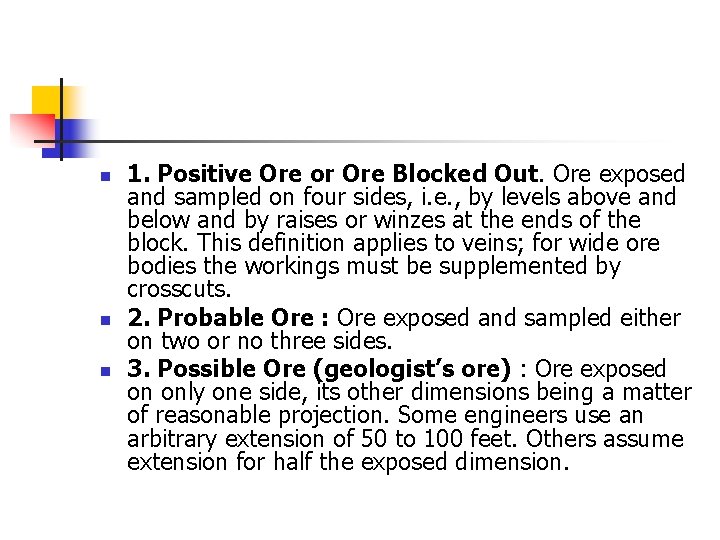
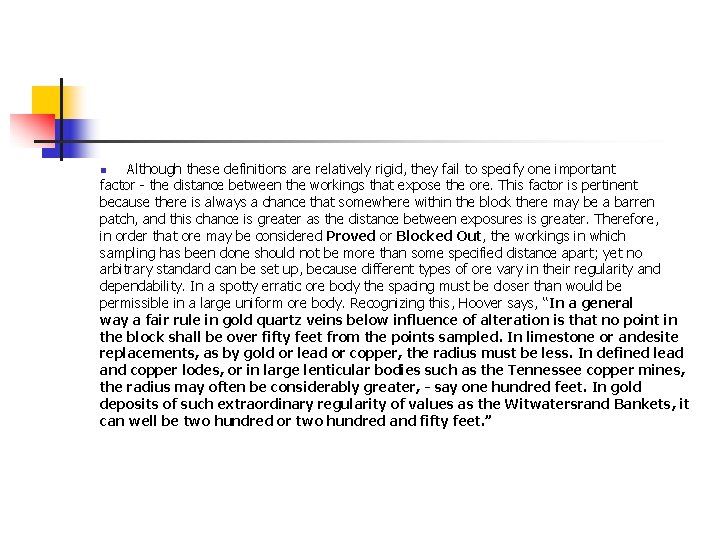
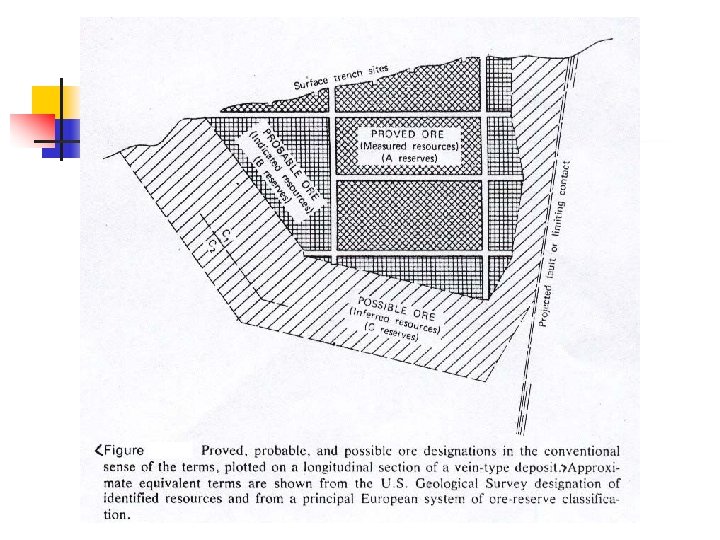
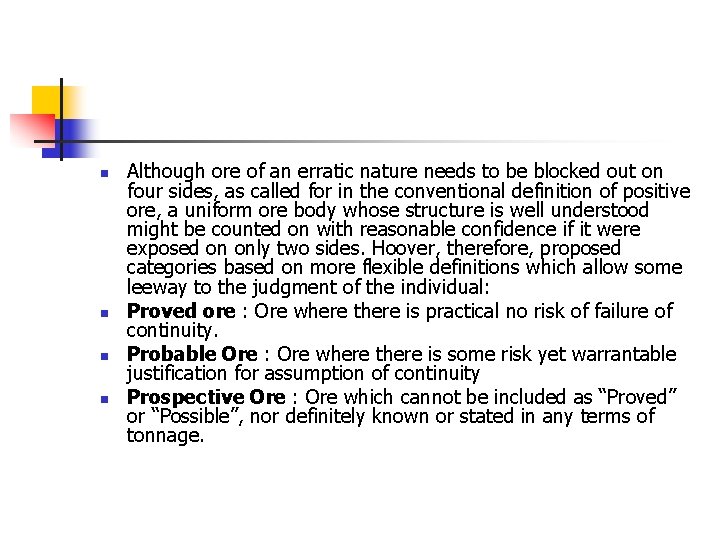
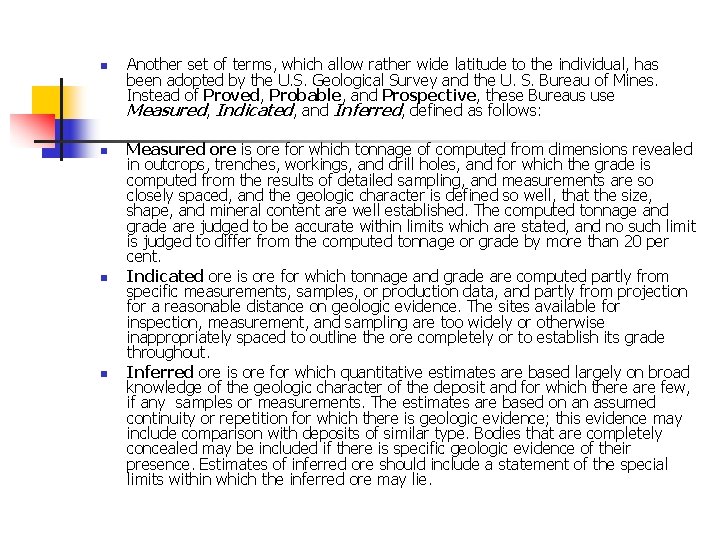
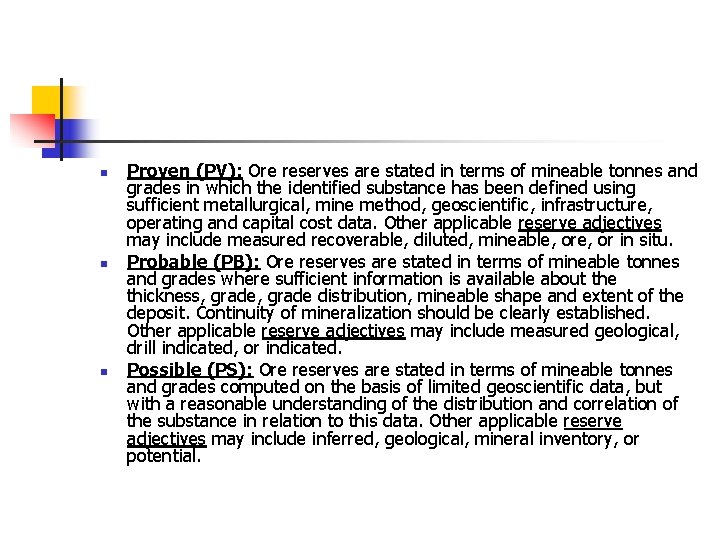
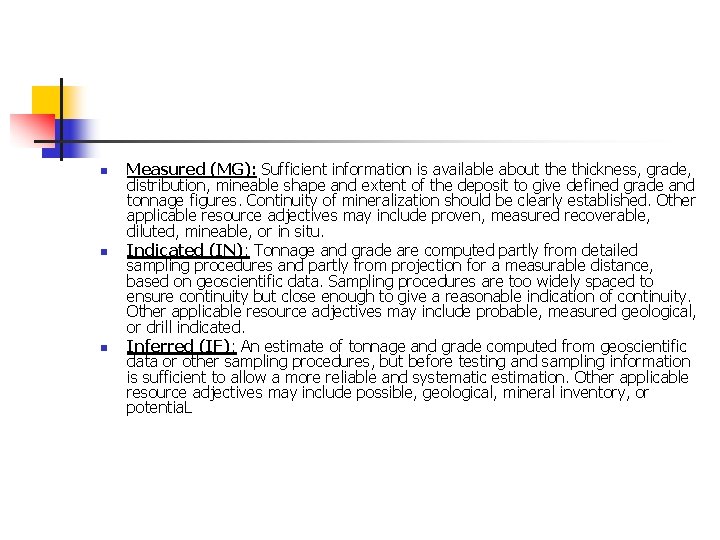
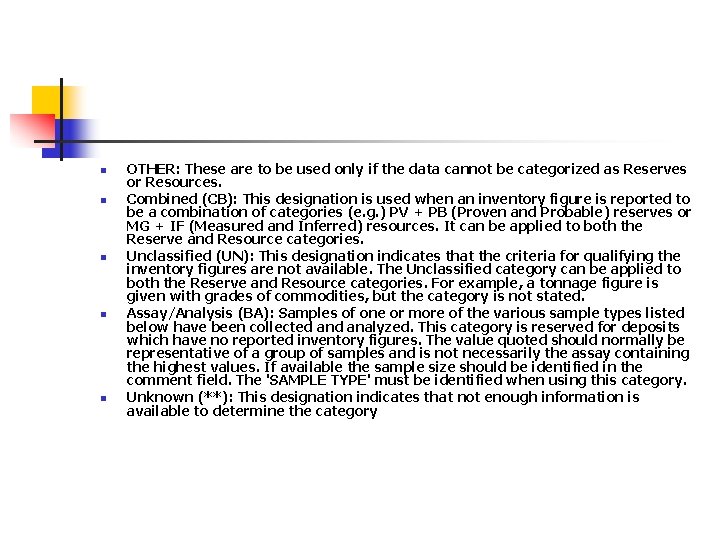
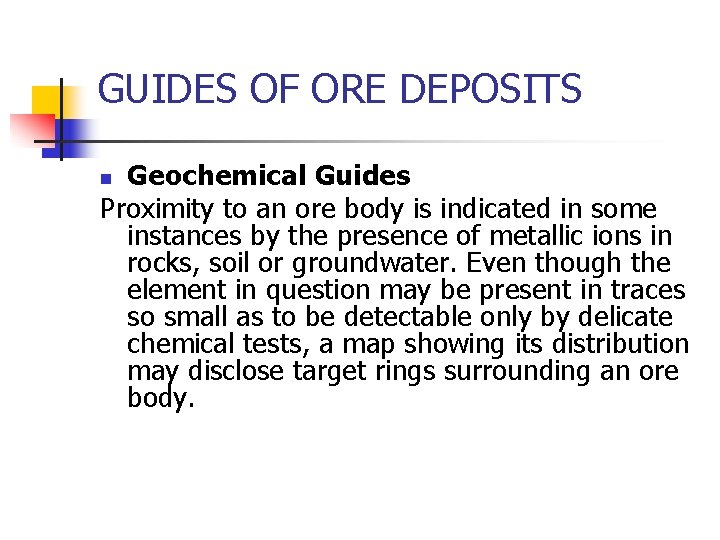
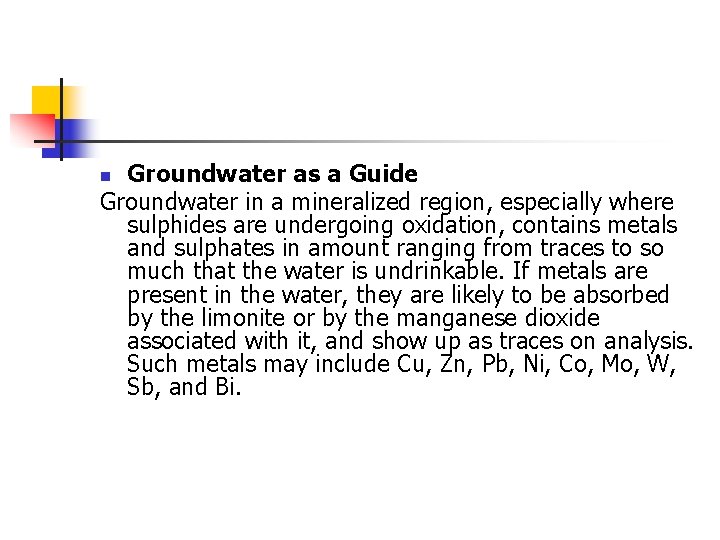
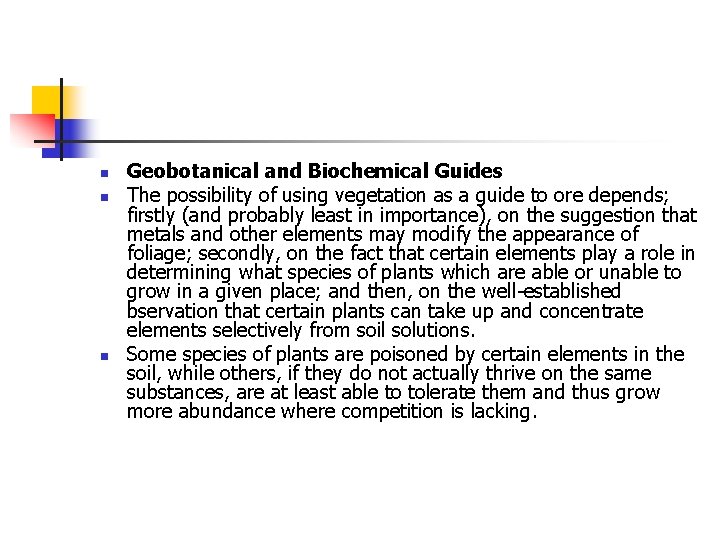
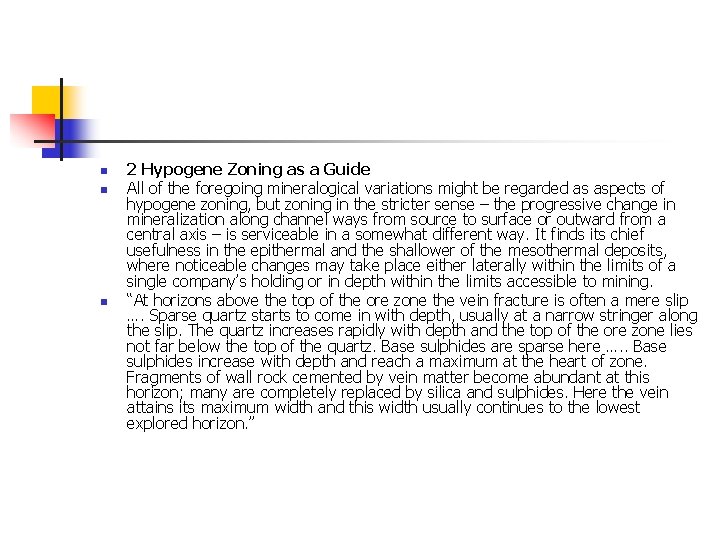
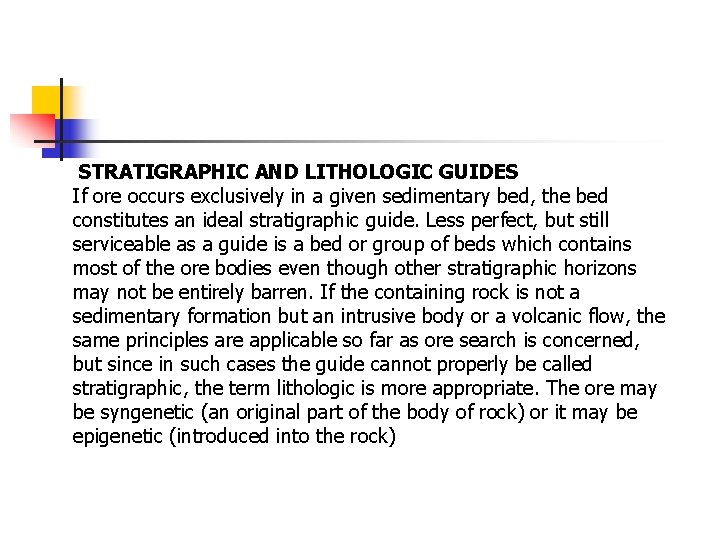
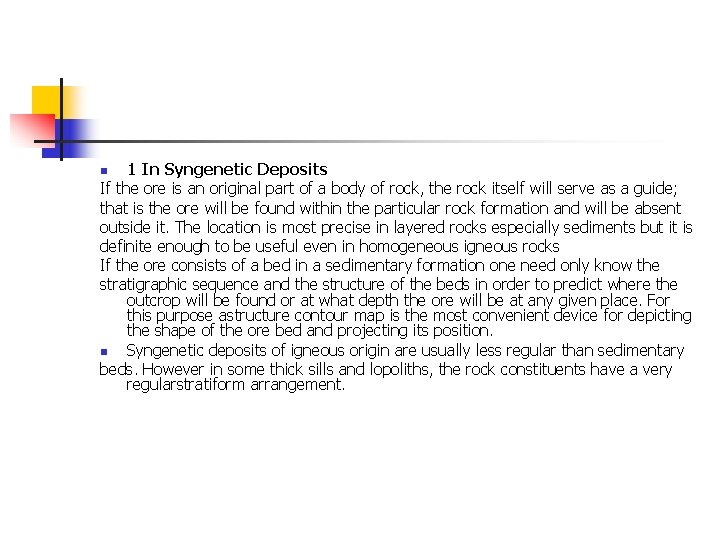
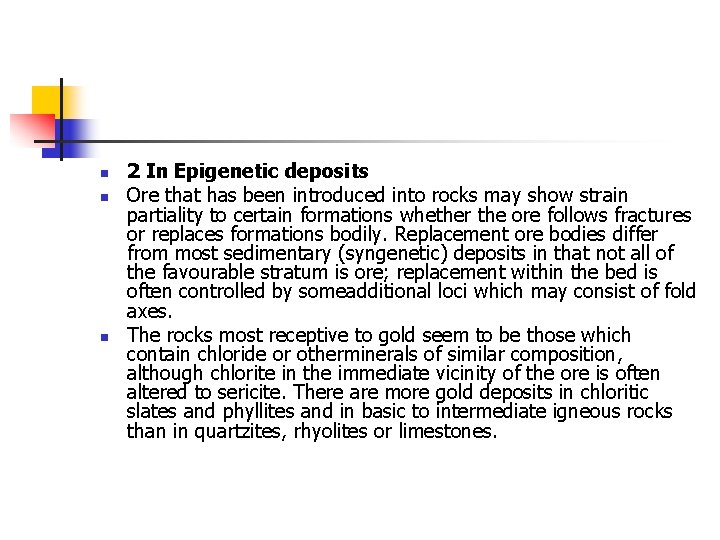
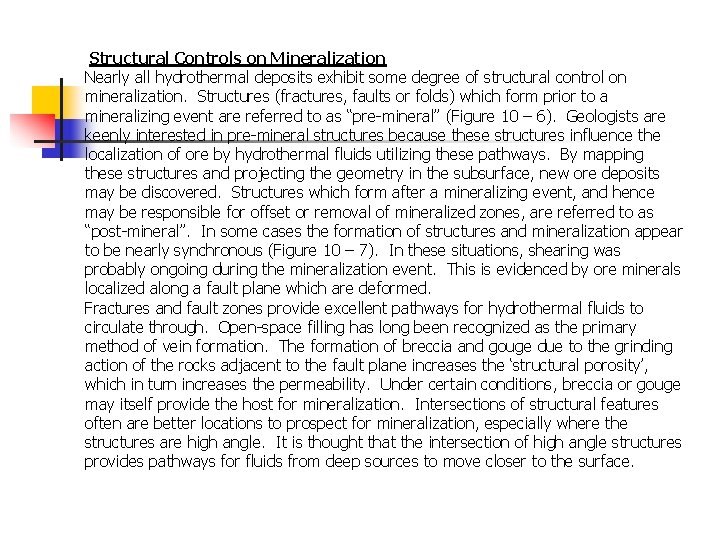
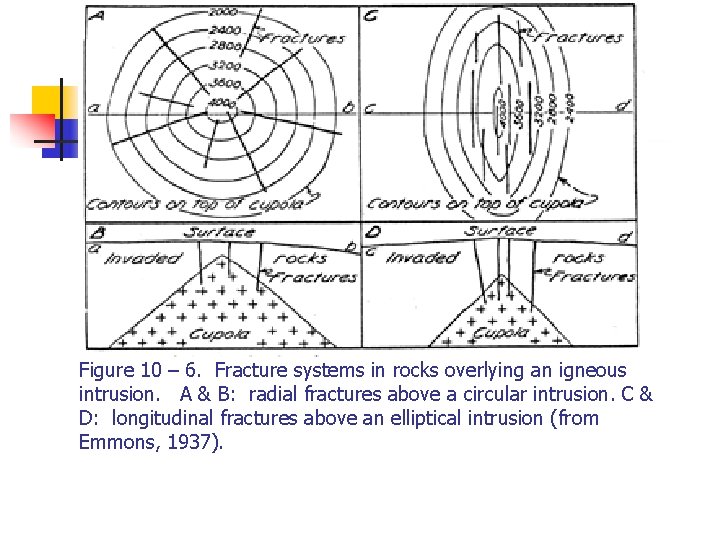
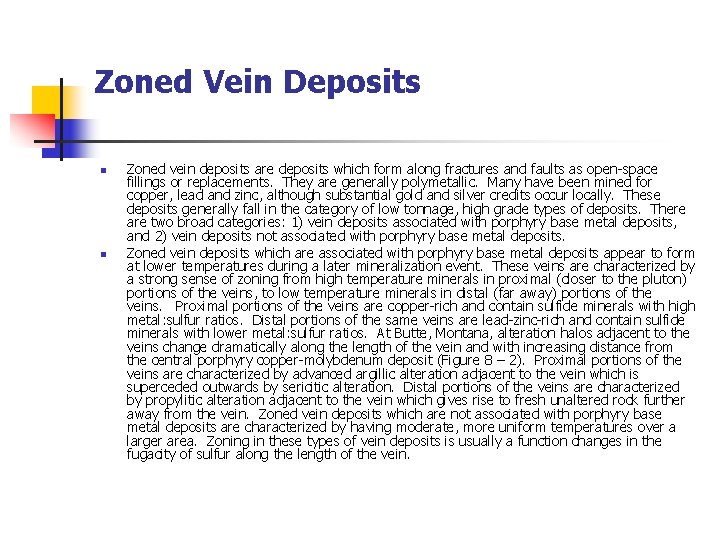
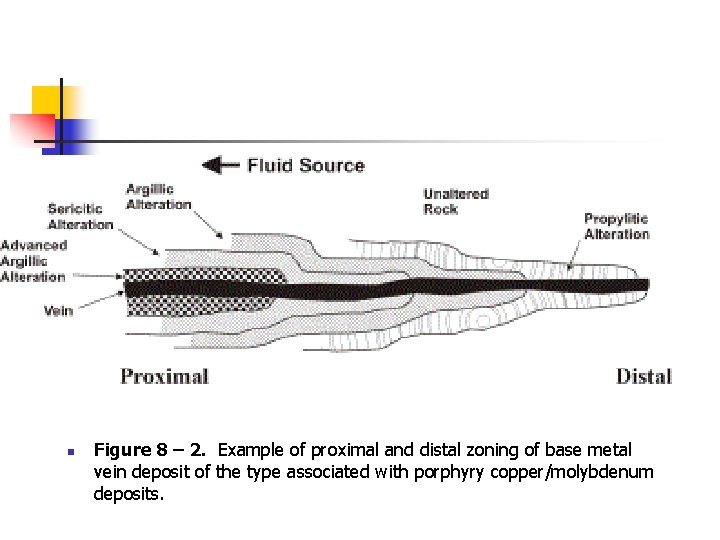
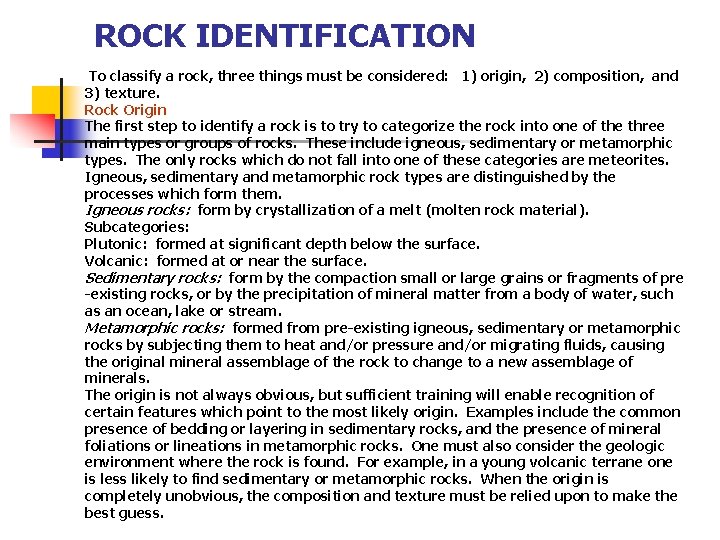
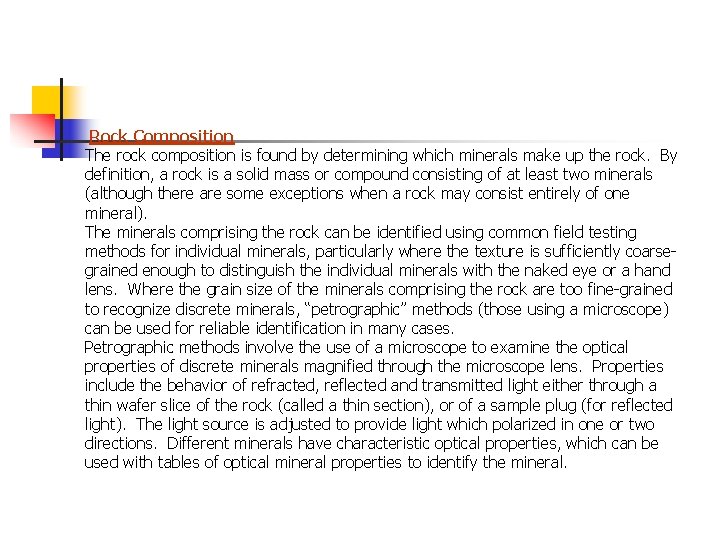
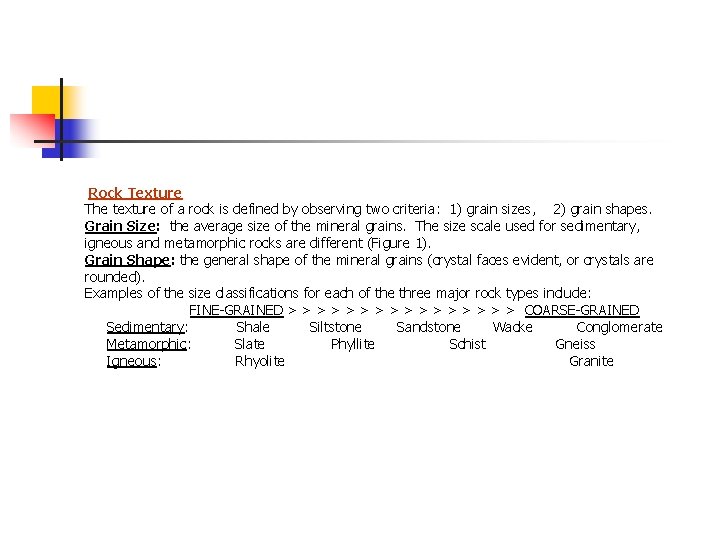
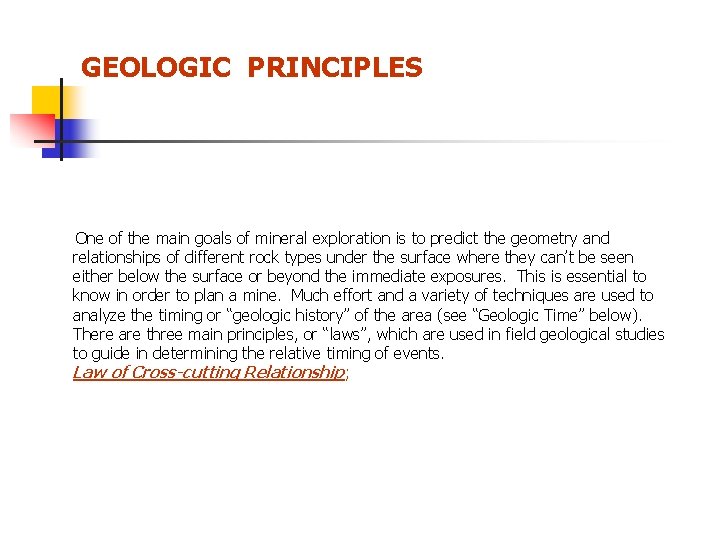
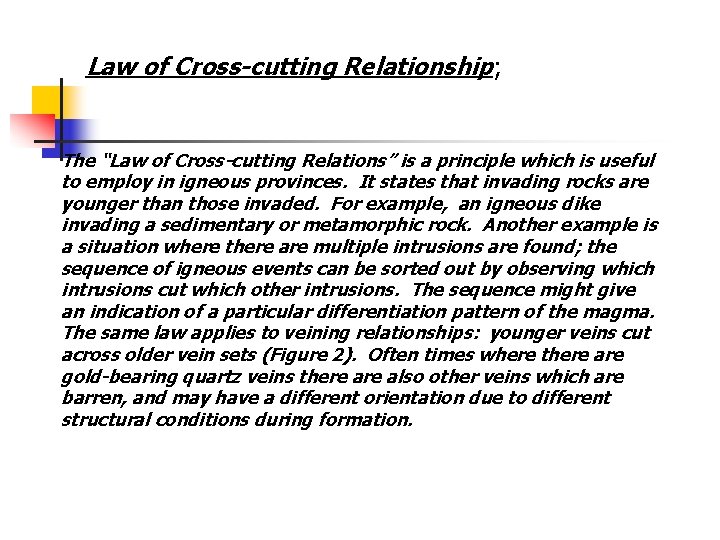
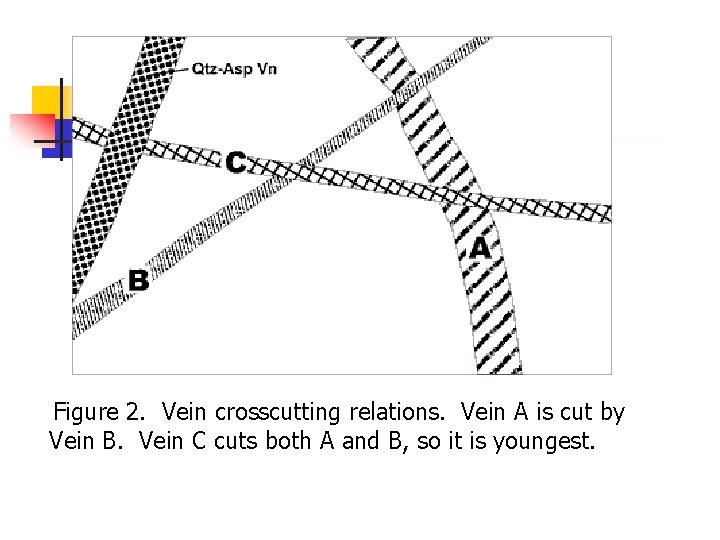
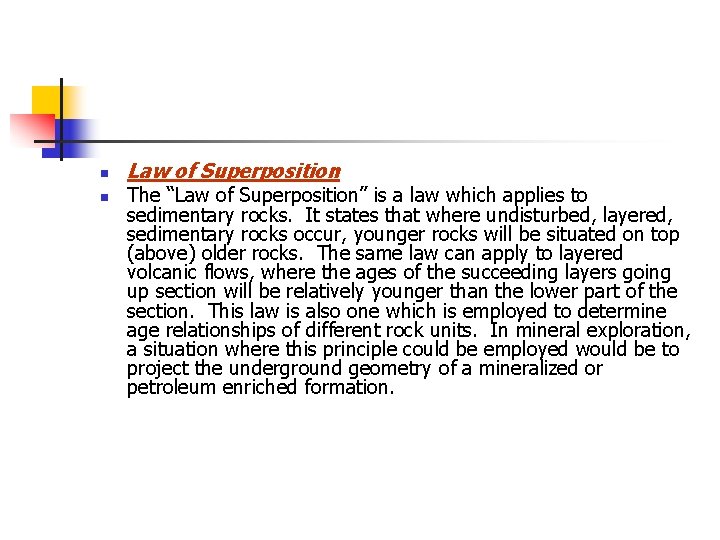
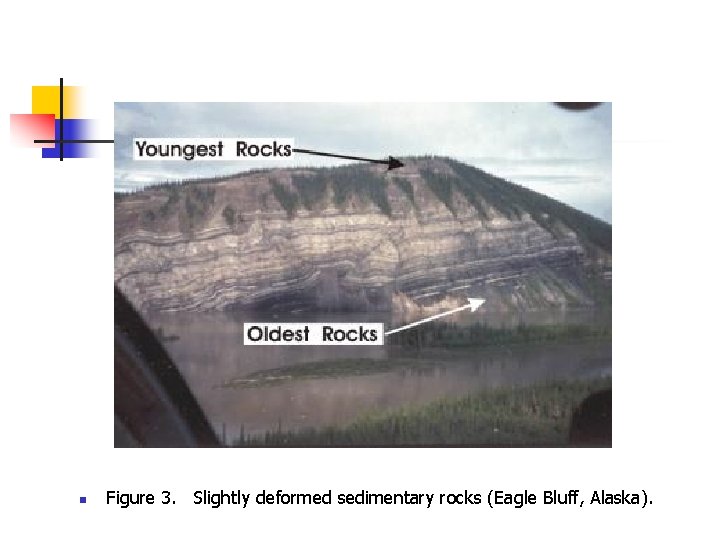
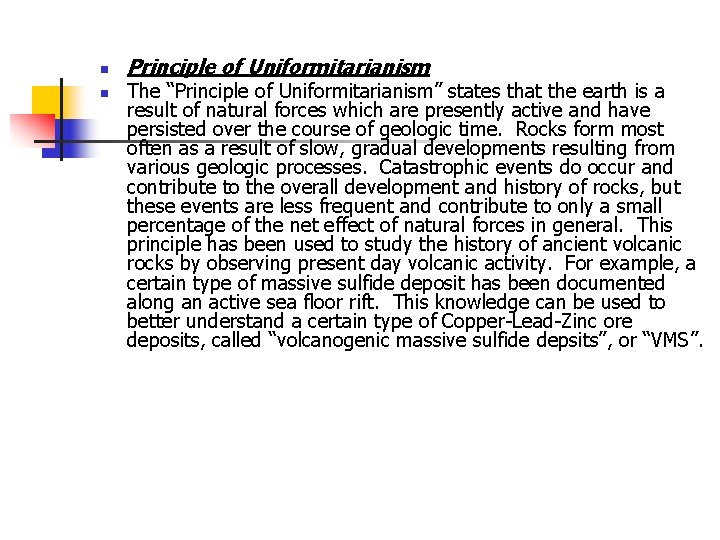
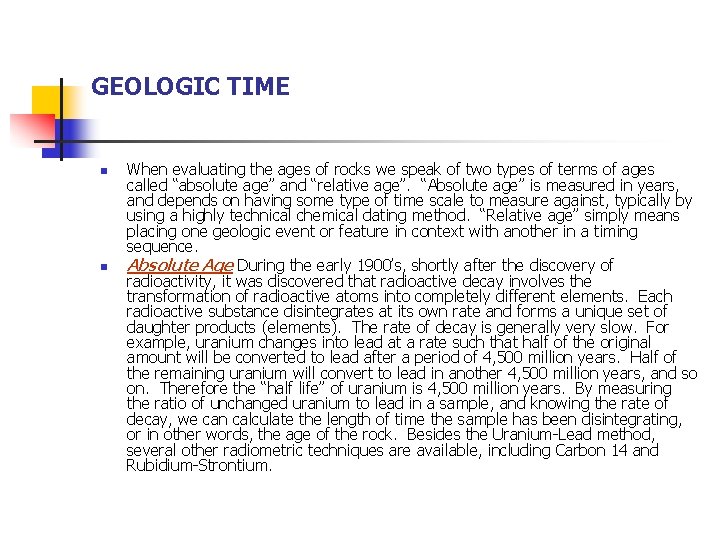
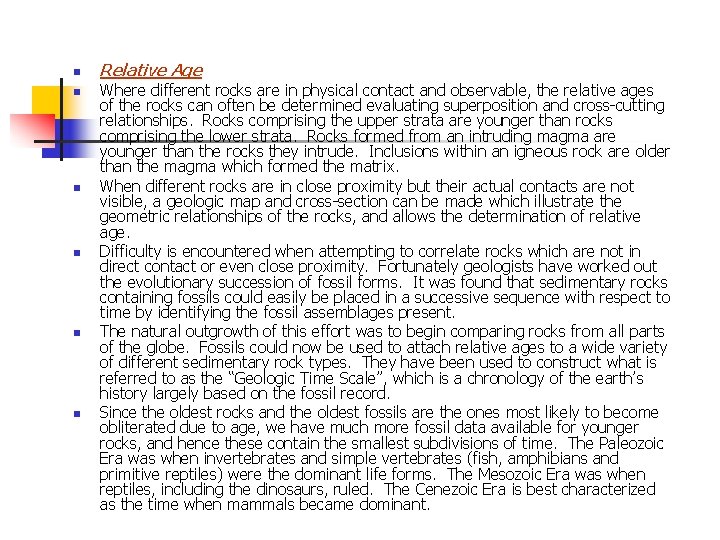
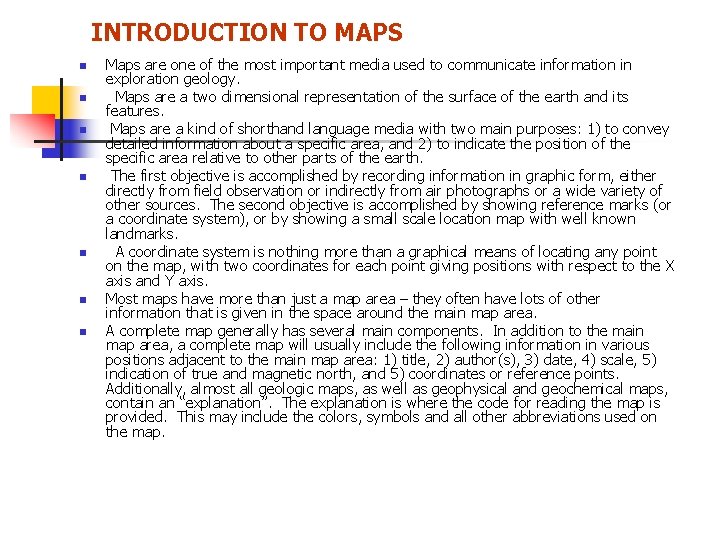
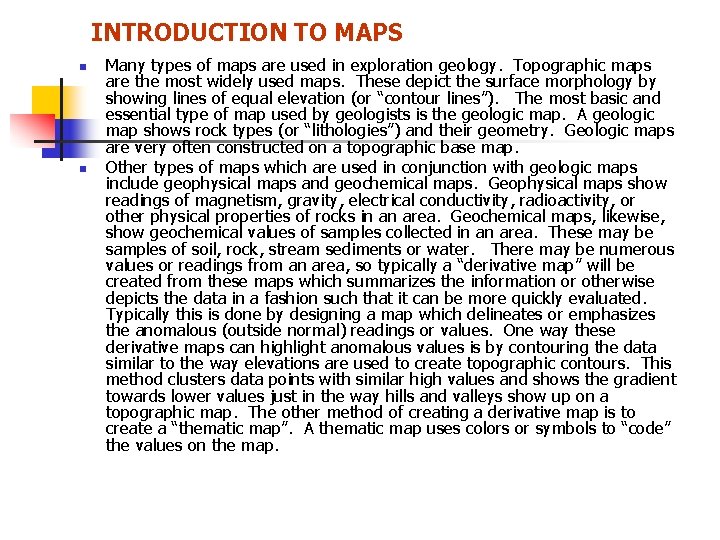
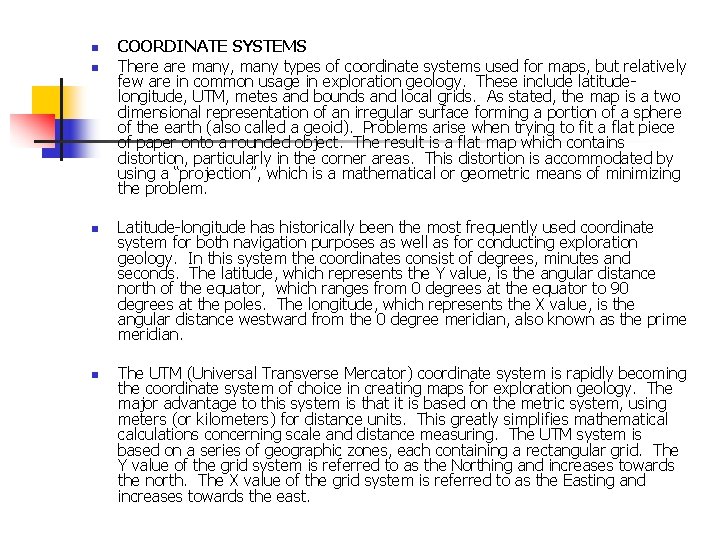
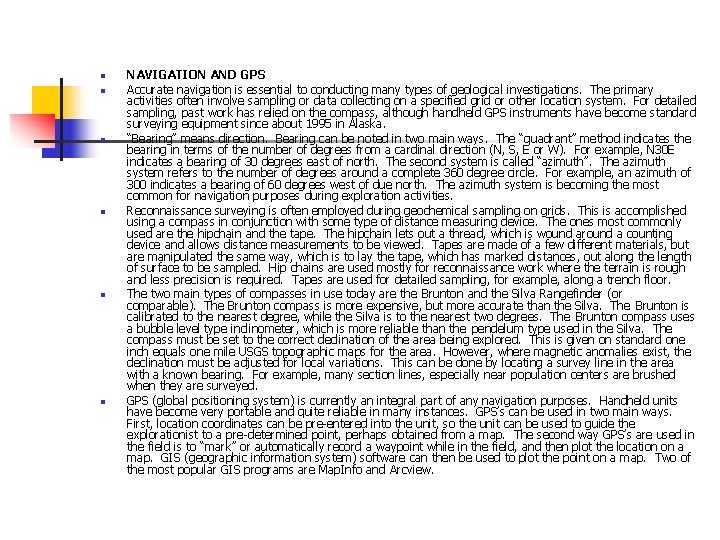
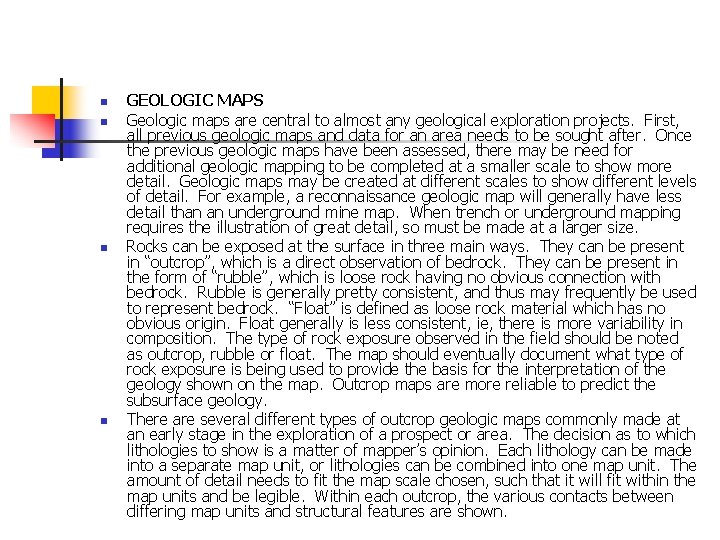
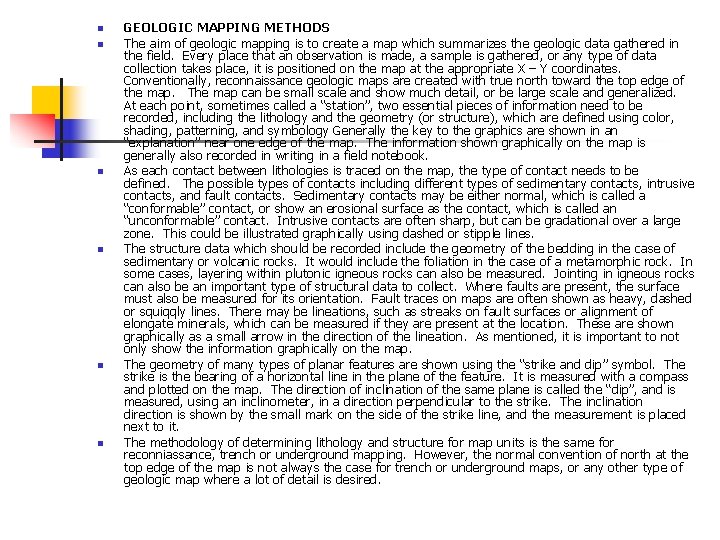
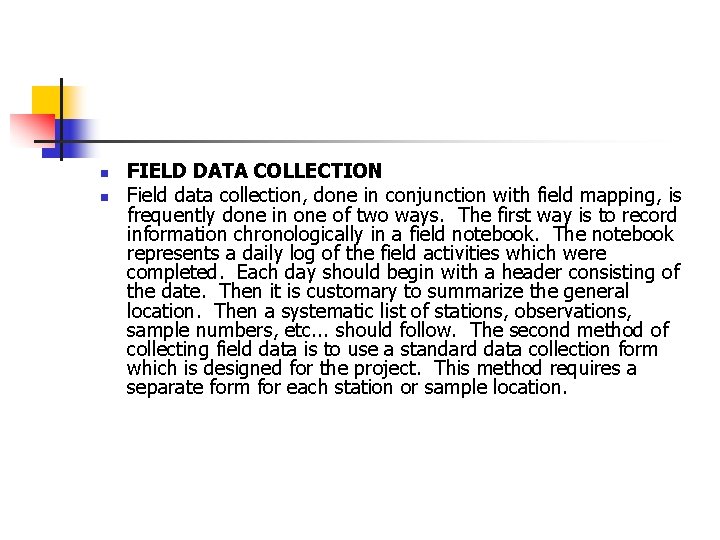
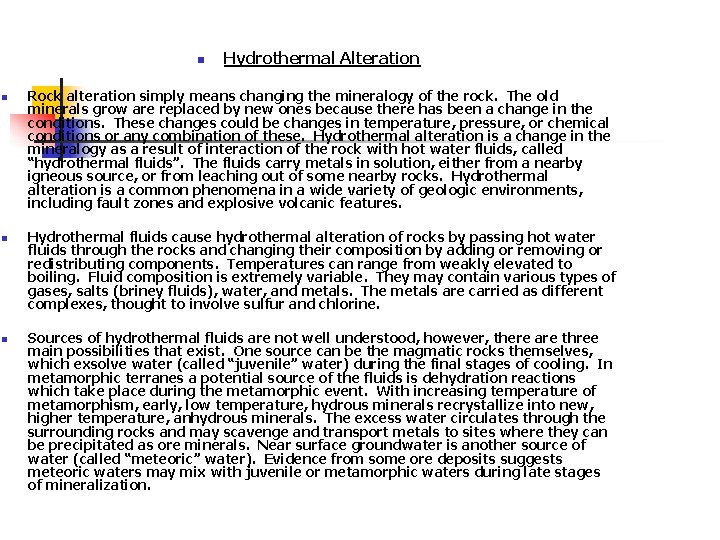
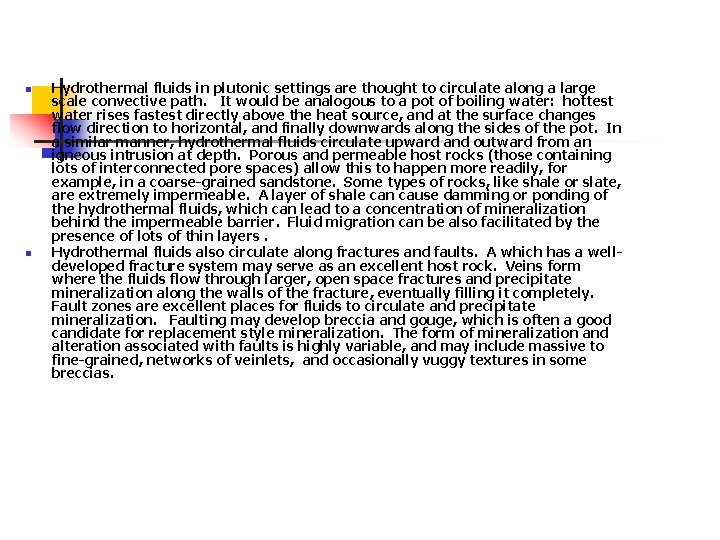
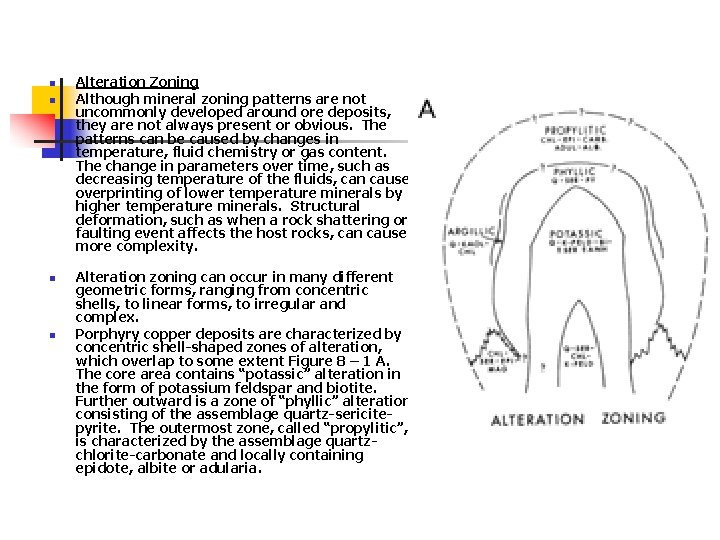
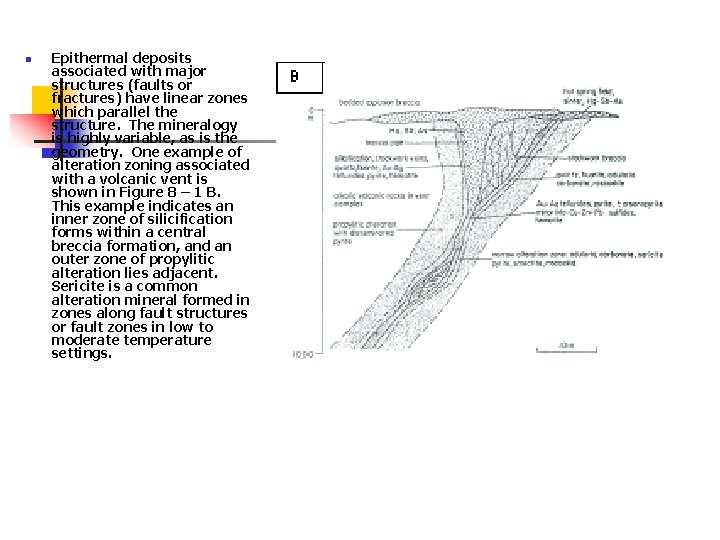
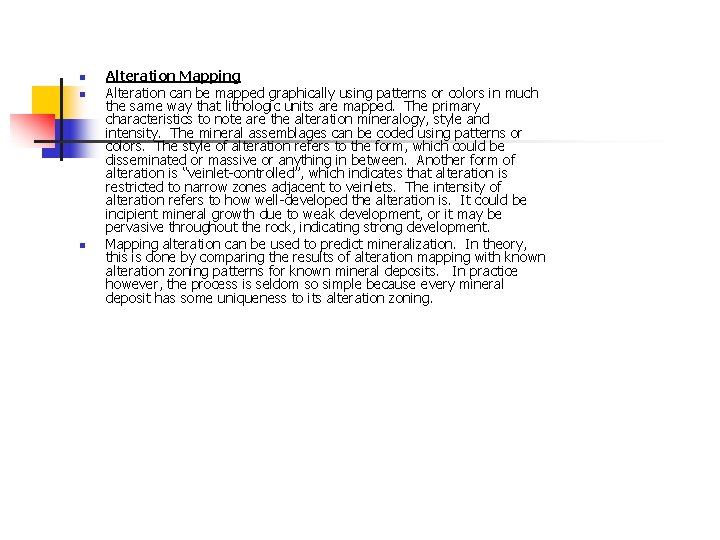
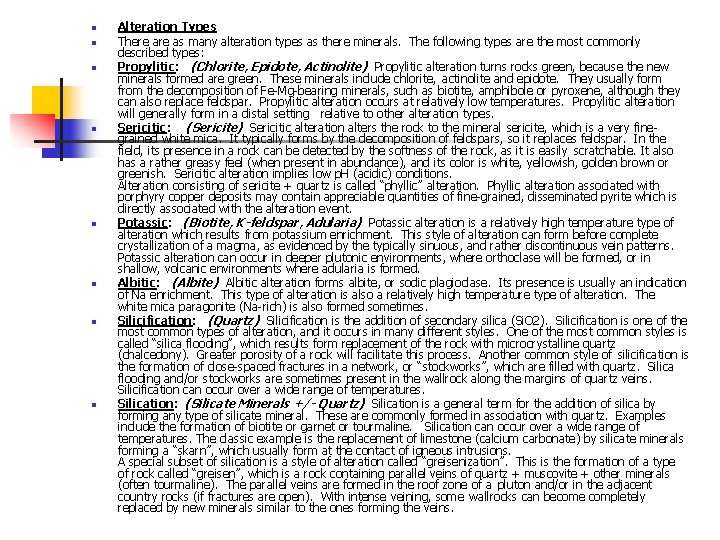
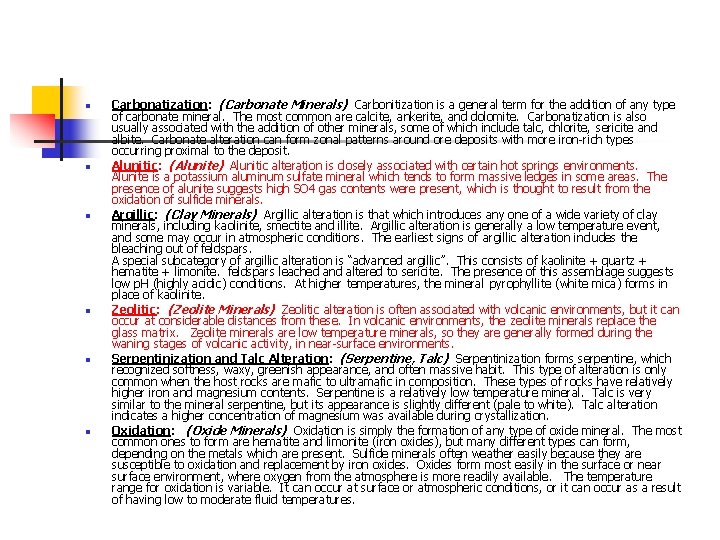
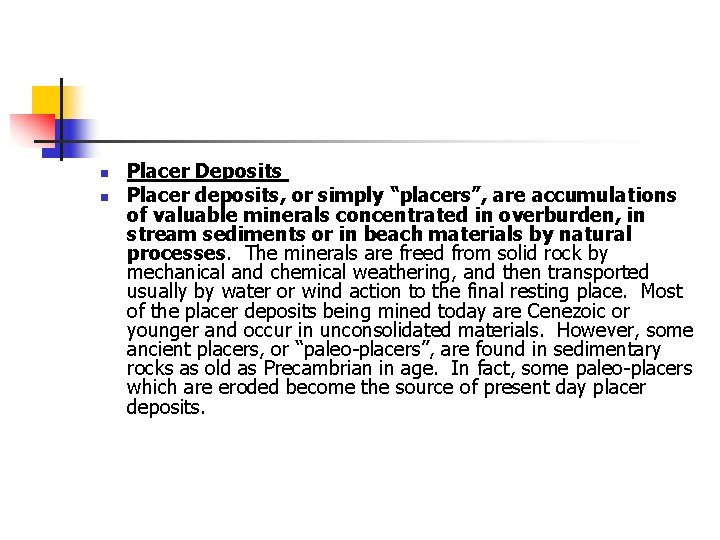
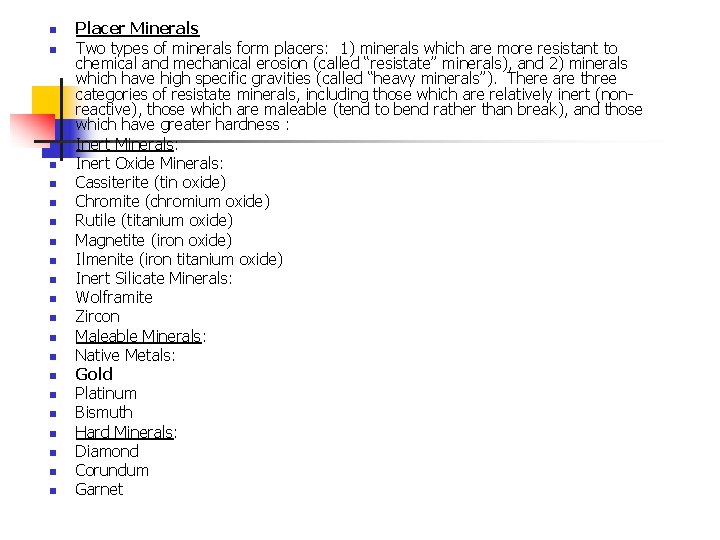
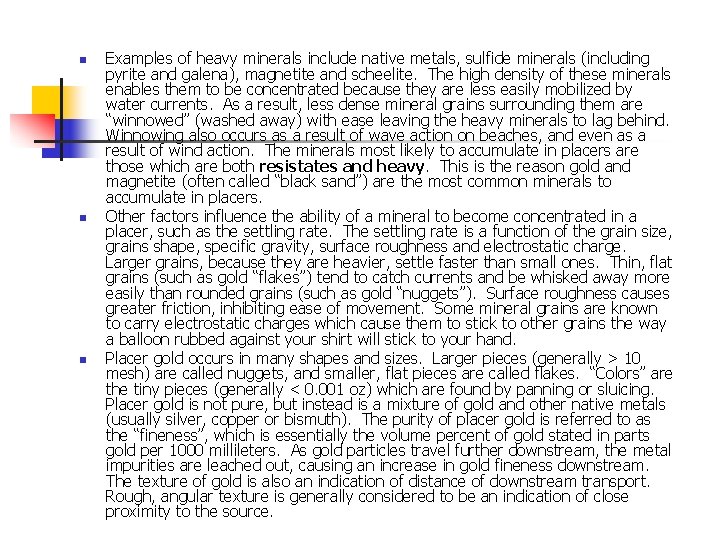
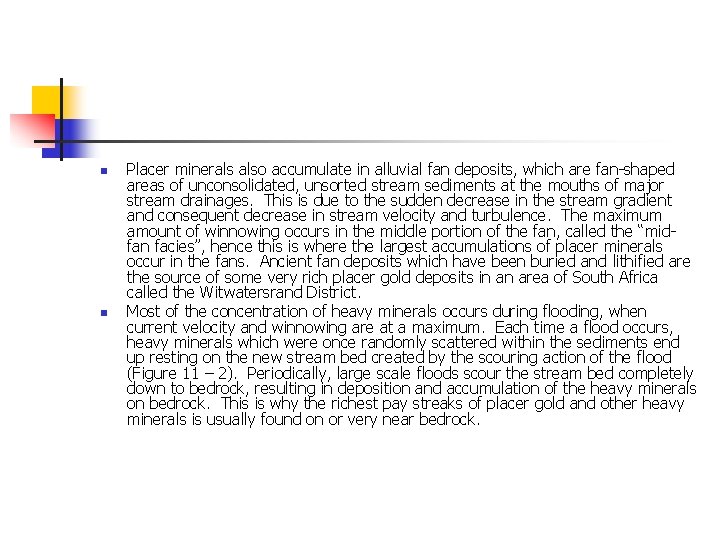
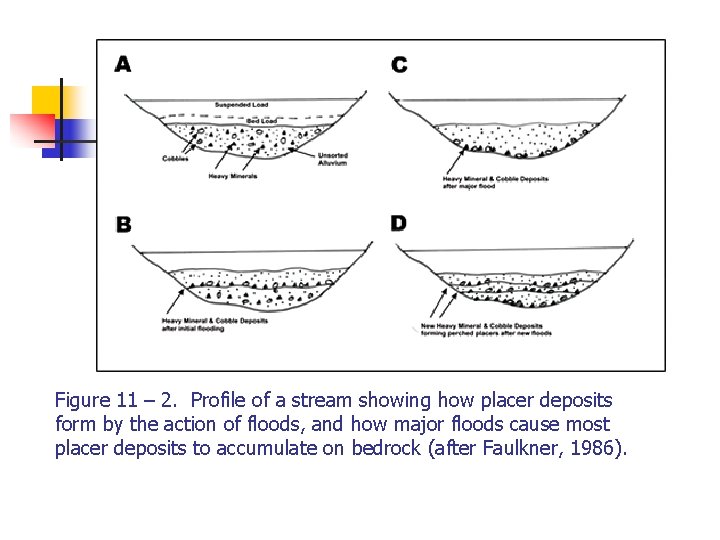
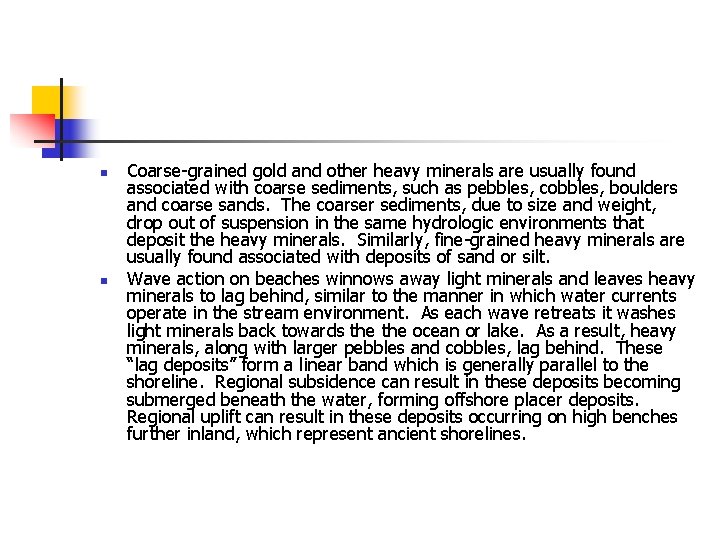
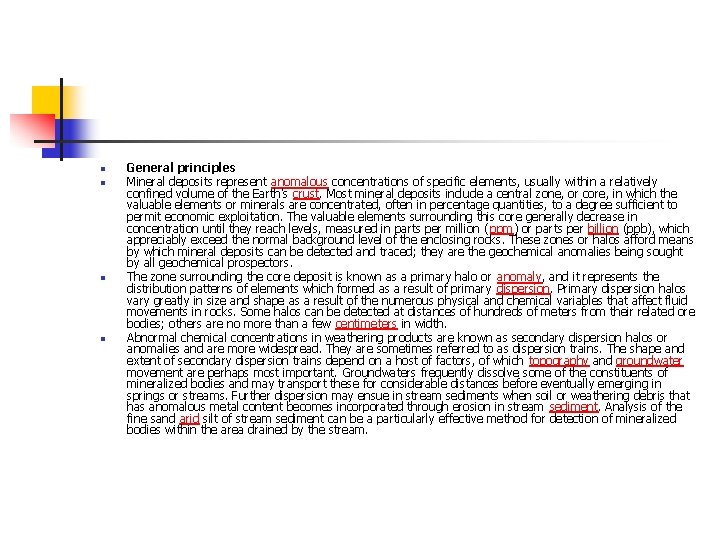
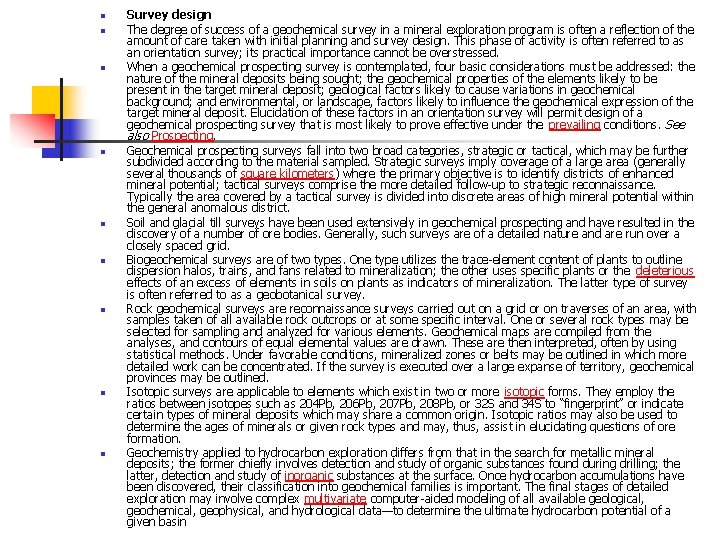
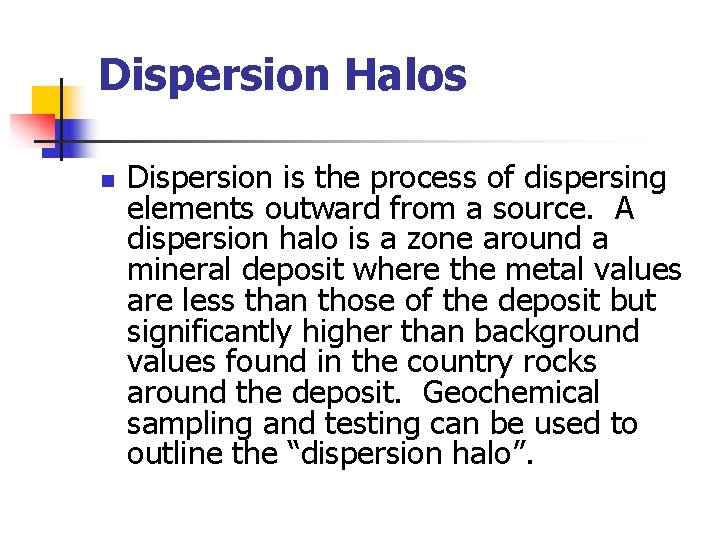
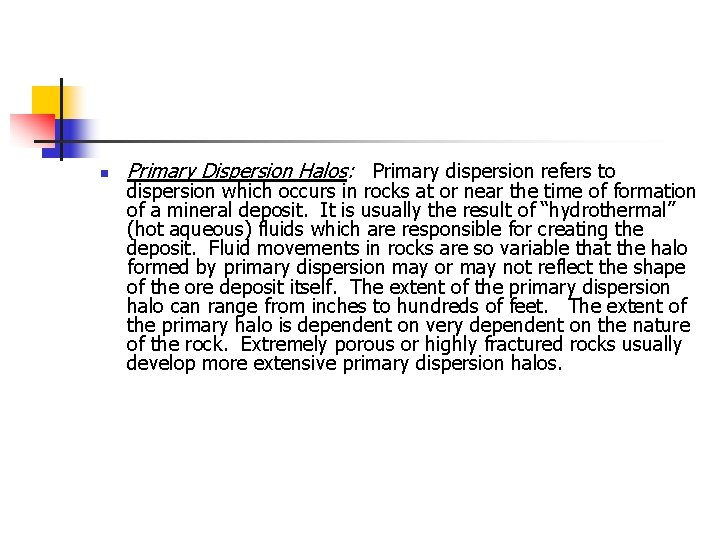
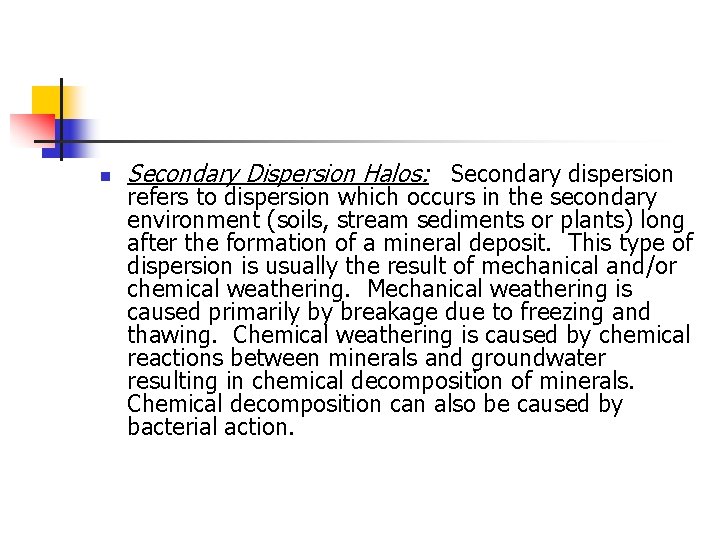
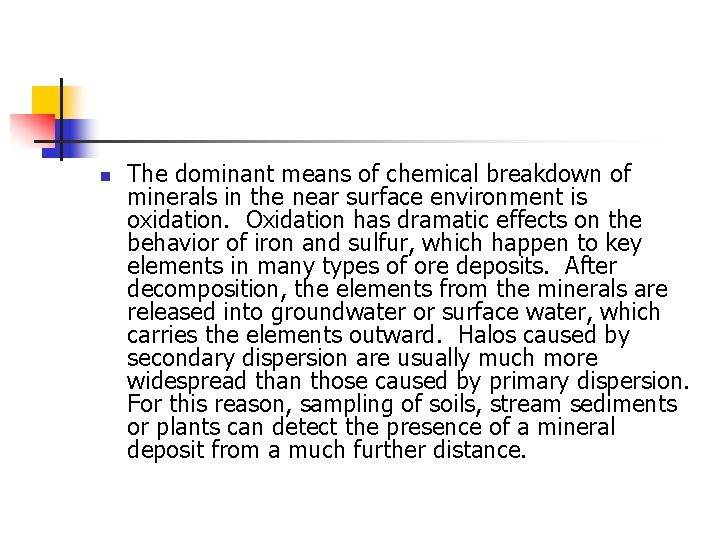
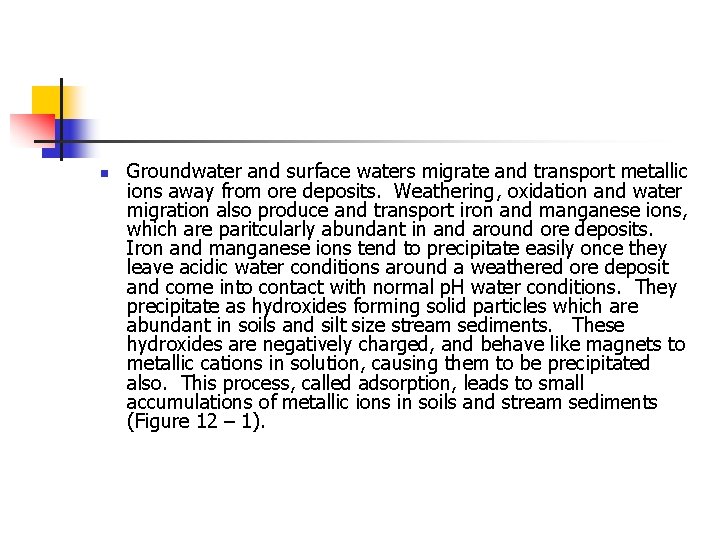
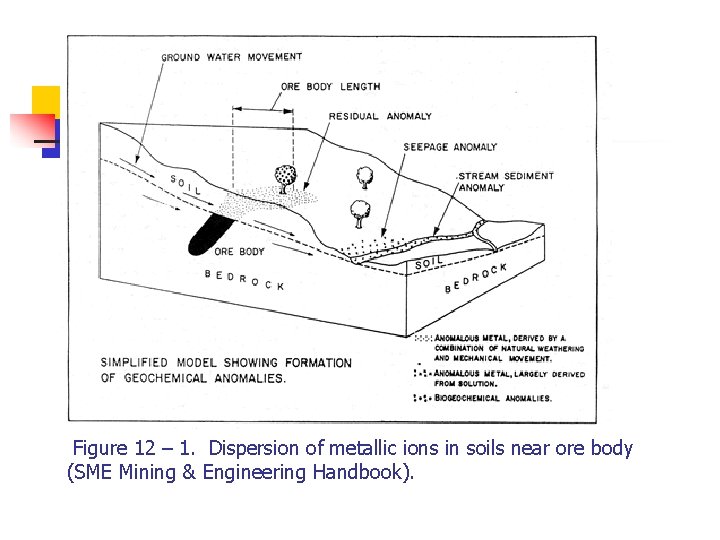
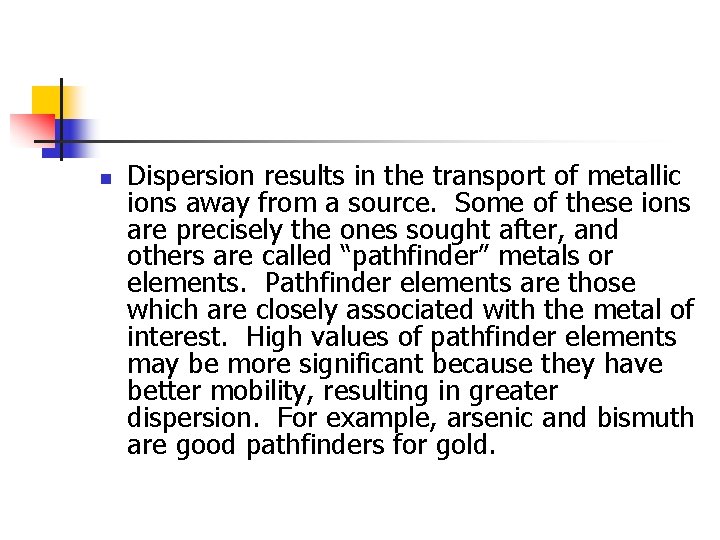
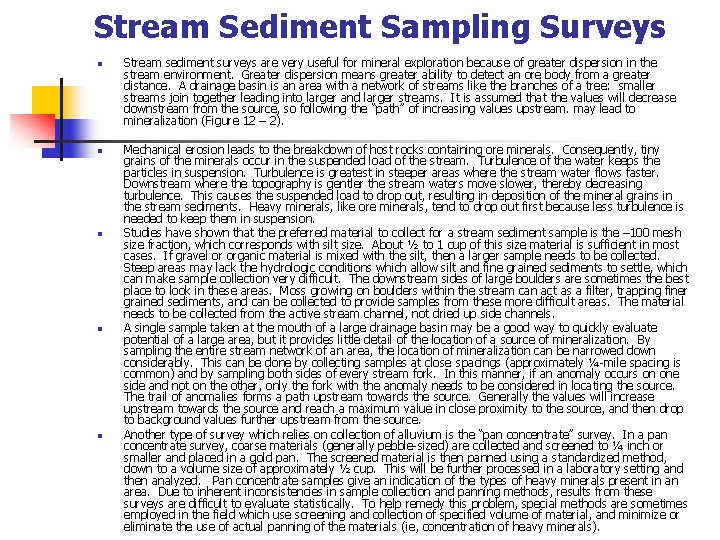
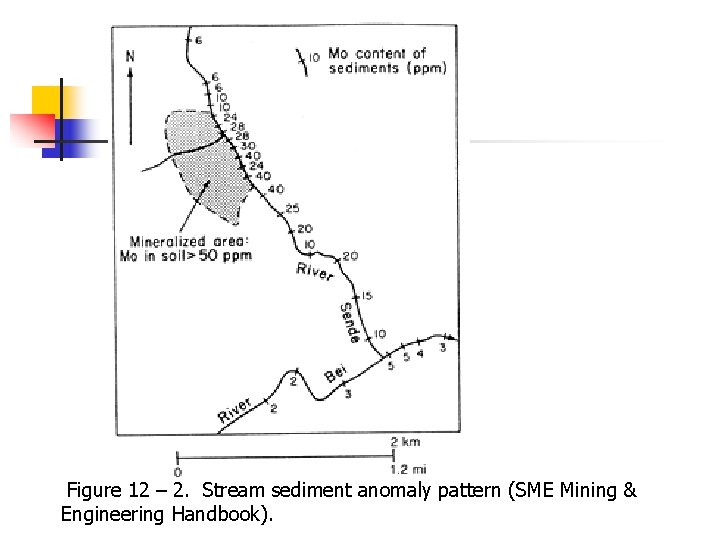
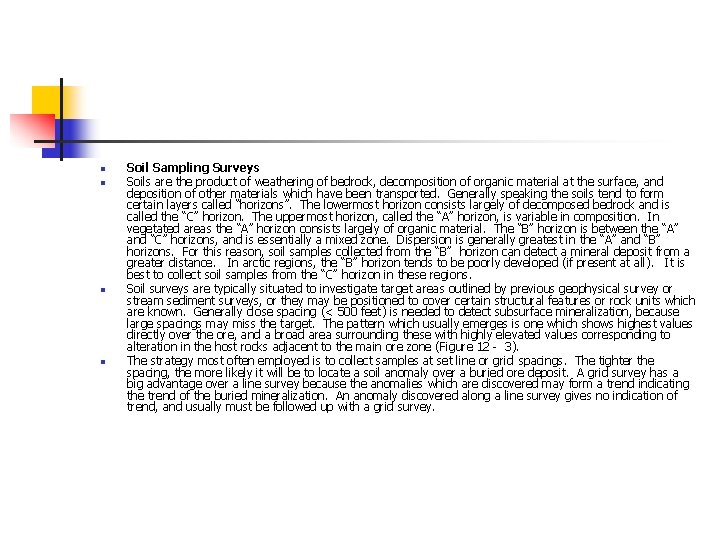
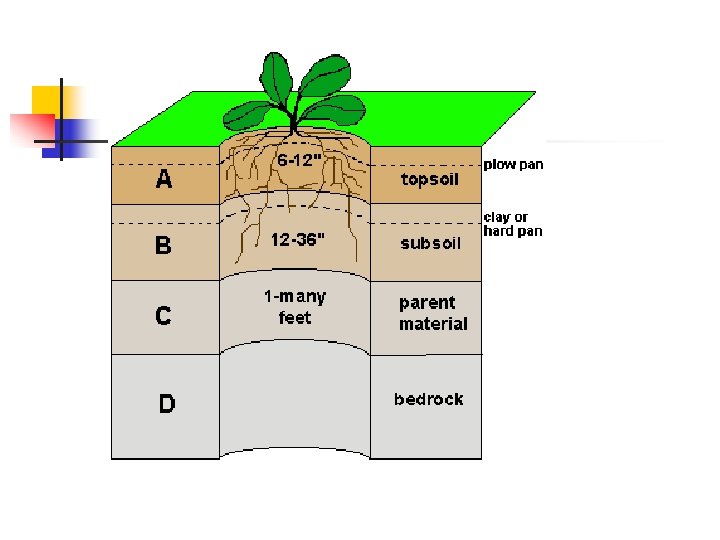
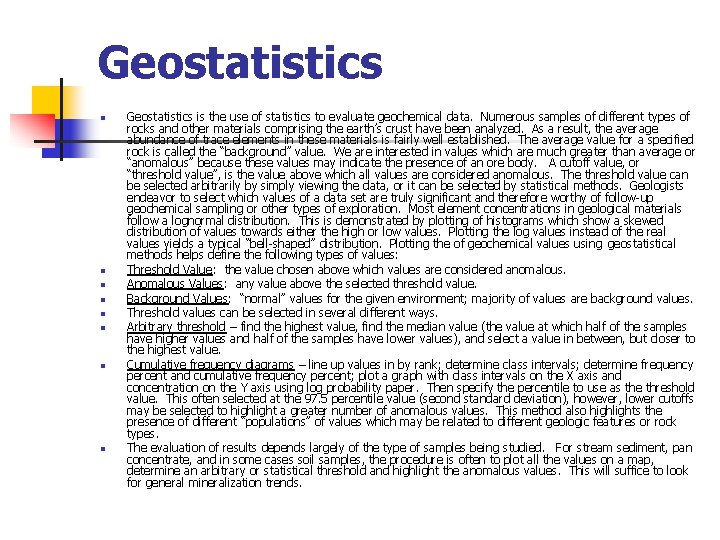
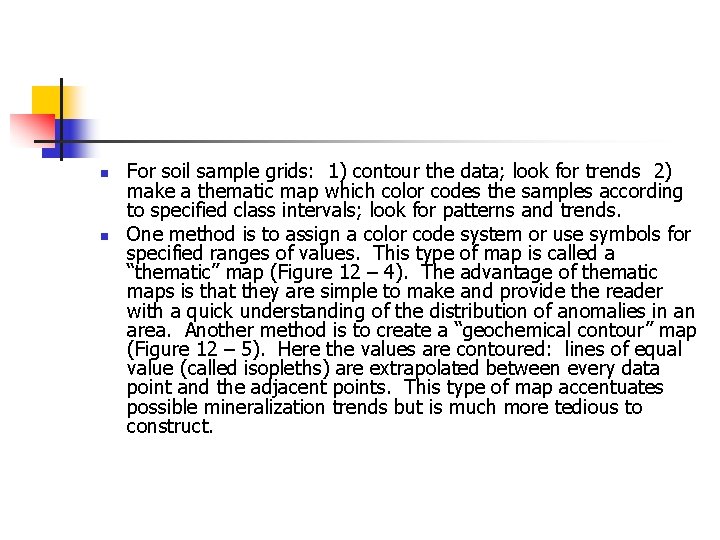
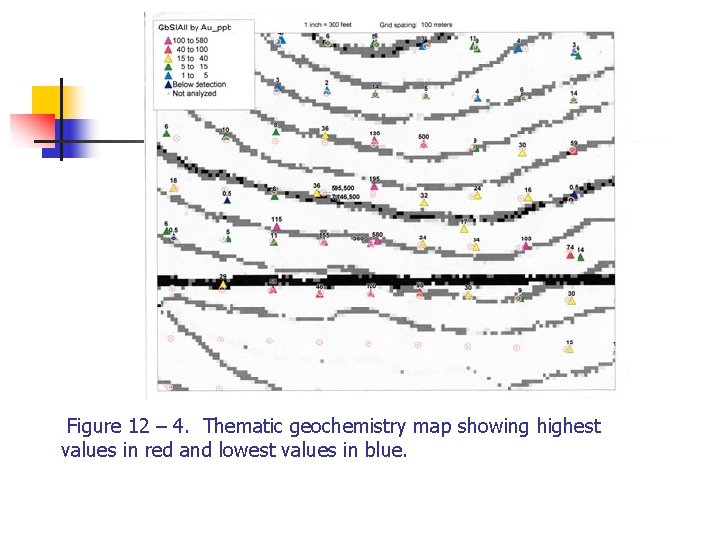
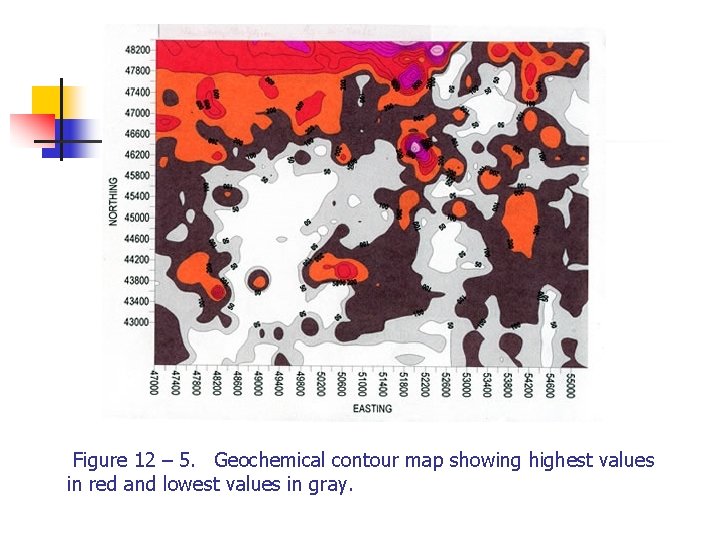
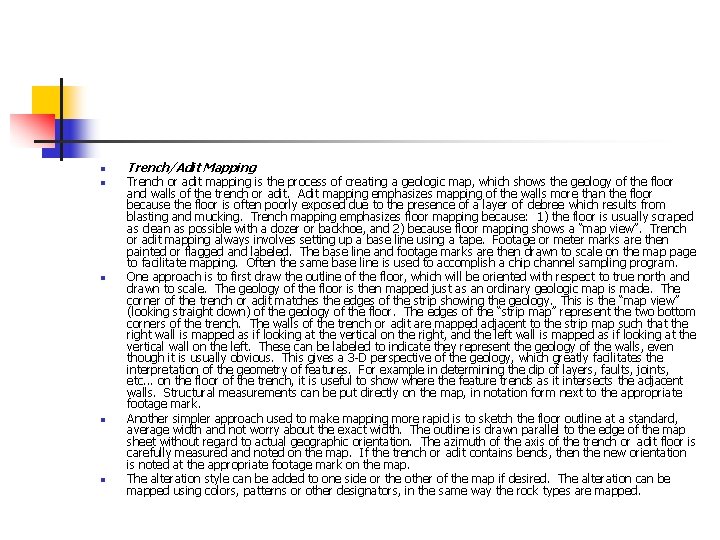
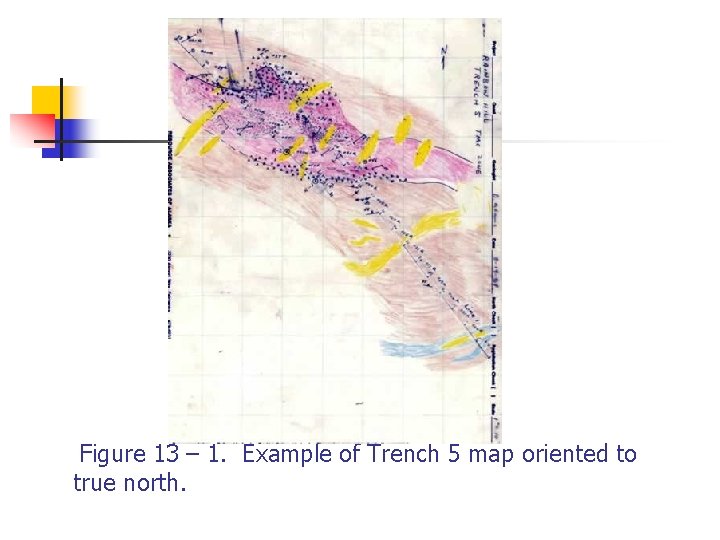
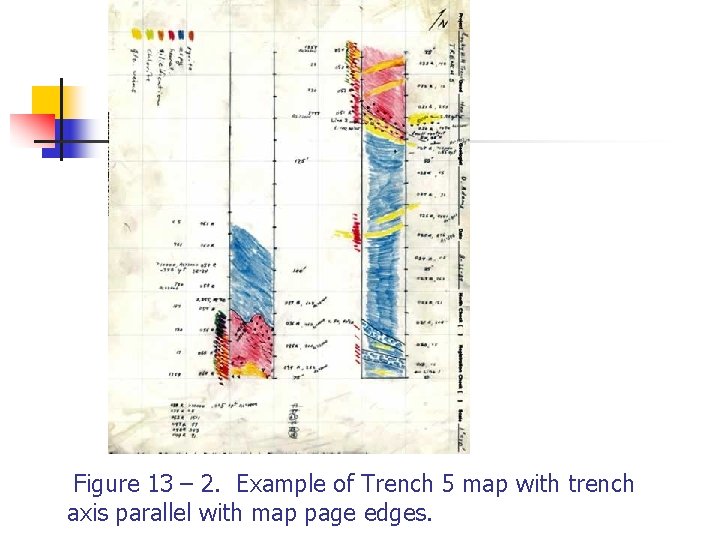
- Slides: 97
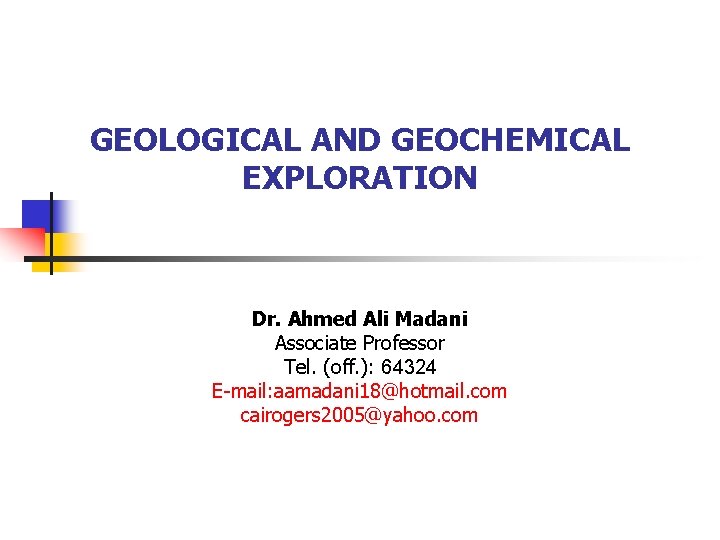
GEOLOGICAL AND GEOCHEMICAL EXPLORATION Dr. Ahmed Ali Madani Associate Professor Tel. (off. ): 64324 E-mail: aamadani 18@hotmail. com cairogers 2005@yahoo. com
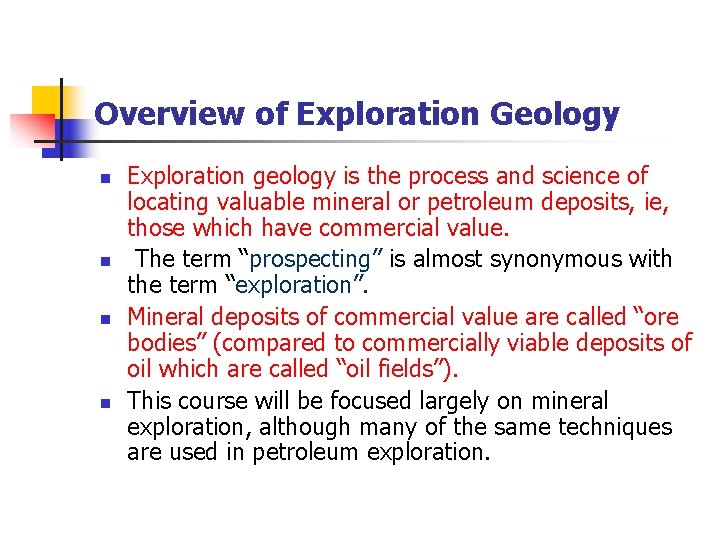
Overview of Exploration Geology n n Exploration geology is the process and science of locating valuable mineral or petroleum deposits, ie, those which have commercial value. The term “prospecting” is almost synonymous with the term “exploration”. Mineral deposits of commercial value are called “ore bodies” (compared to commercially viable deposits of oil which are called “oil fields”). This course will be focused largely on mineral exploration, although many of the same techniques are used in petroleum exploration.
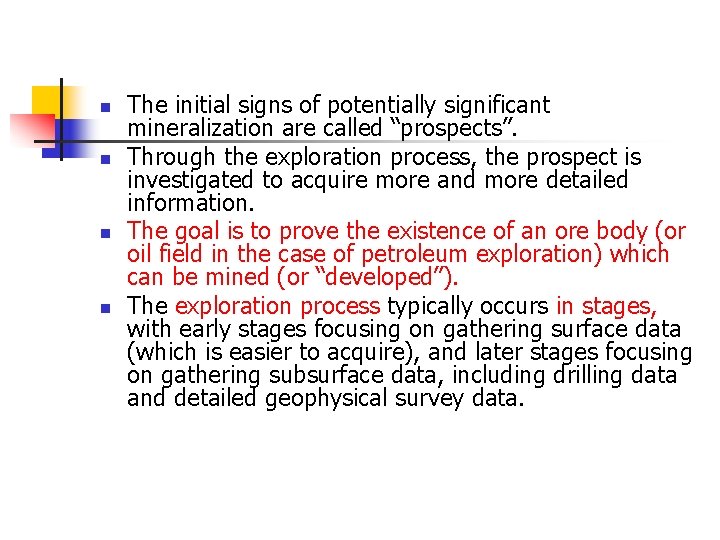
n n The initial signs of potentially significant mineralization are called “prospects”. Through the exploration process, the prospect is investigated to acquire more and more detailed information. The goal is to prove the existence of an ore body (or oil field in the case of petroleum exploration) which can be mined (or “developed”). The exploration process typically occurs in stages, with early stages focusing on gathering surface data (which is easier to acquire), and later stages focusing on gathering subsurface data, including drilling data and detailed geophysical survey data.
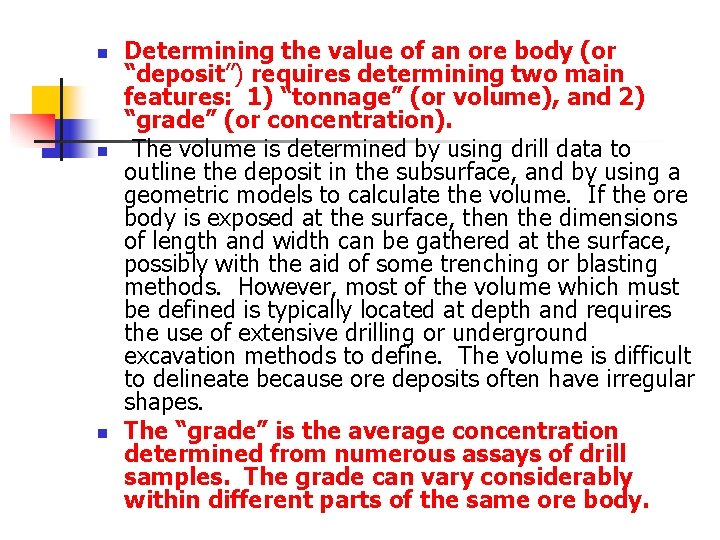
n n n Determining the value of an ore body (or “deposit”) requires determining two main features: 1) “tonnage” (or volume), and 2) “grade” (or concentration). The volume is determined by using drill data to outline the deposit in the subsurface, and by using a geometric models to calculate the volume. If the ore body is exposed at the surface, then the dimensions of length and width can be gathered at the surface, possibly with the aid of some trenching or blasting methods. However, most of the volume which must be defined is typically located at depth and requires the use of extensive drilling or underground excavation methods to define. The volume is difficult to delineate because ore deposits often have irregular shapes. The “grade” is the average concentration determined from numerous assays of drill samples. The grade can vary considerably within different parts of the same ore body.
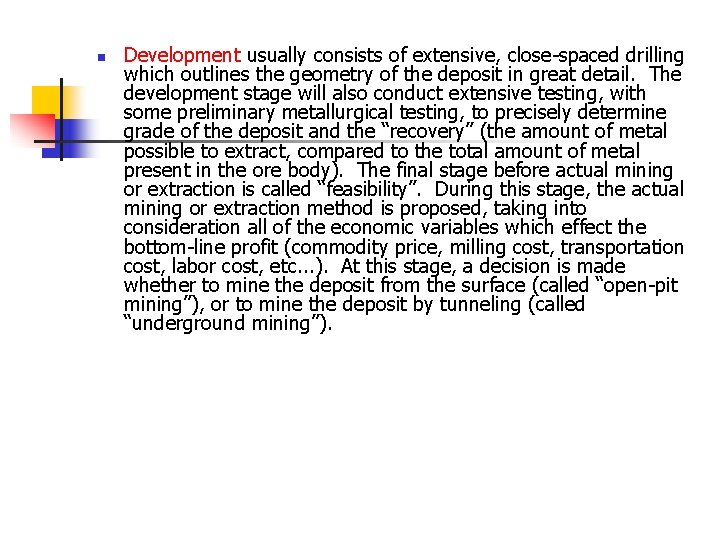
n Development usually consists of extensive, close-spaced drilling which outlines the geometry of the deposit in great detail. The development stage will also conduct extensive testing, with some preliminary metallurgical testing, to precisely determine grade of the deposit and the “recovery” (the amount of metal possible to extract, compared to the total amount of metal present in the ore body). The final stage before actual mining or extraction is called “feasibility”. During this stage, the actual mining or extraction method is proposed, taking into consideration all of the economic variables which effect the bottom-line profit (commodity price, milling cost, transportation cost, labor cost, etc. . . ). At this stage, a decision is made whether to mine the deposit from the surface (called “open-pit mining”), or to mine the deposit by tunneling (called “underground mining”).
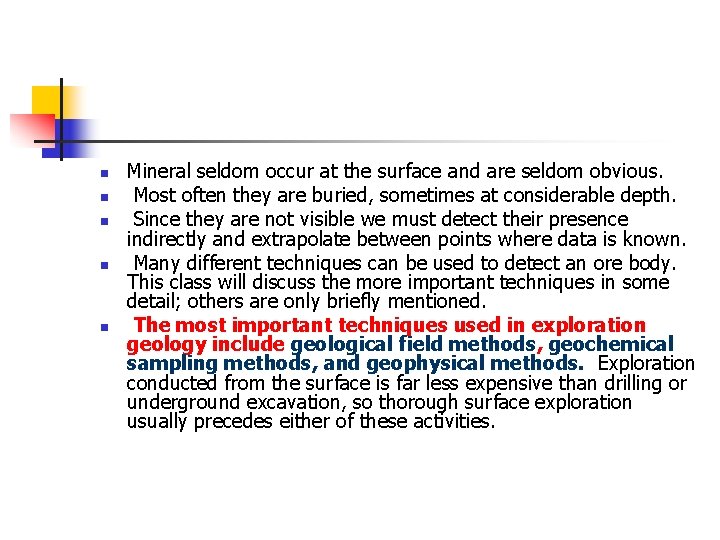
n n n Mineral seldom occur at the surface and are seldom obvious. Most often they are buried, sometimes at considerable depth. Since they are not visible we must detect their presence indirectly and extrapolate between points where data is known. Many different techniques can be used to detect an ore body. This class will discuss the more important techniques in some detail; others are only briefly mentioned. The most important techniques used in exploration geology include geological field methods, geochemical sampling methods, and geophysical methods. Exploration conducted from the surface is far less expensive than drilling or underground excavation, so thorough surface exploration usually precedes either of these activities.
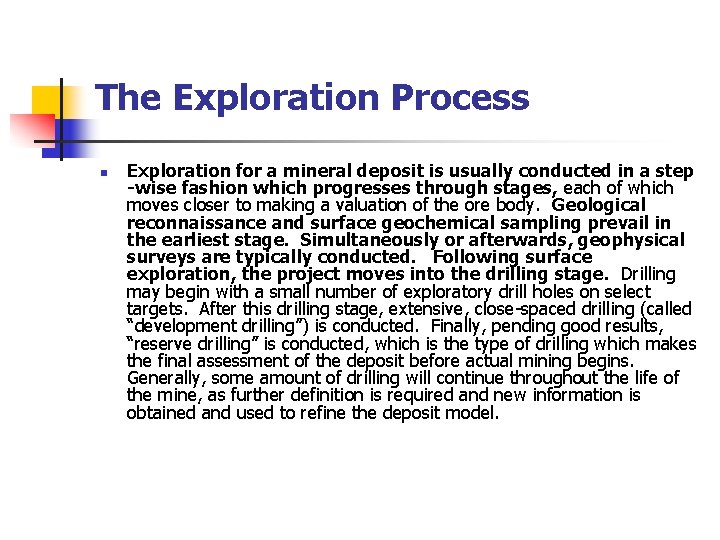
The Exploration Process n Exploration for a mineral deposit is usually conducted in a step -wise fashion which progresses through stages, each of which moves closer to making a valuation of the ore body. Geological reconnaissance and surface geochemical sampling prevail in the earliest stage. Simultaneously or afterwards, geophysical surveys are typically conducted. Following surface exploration, the project moves into the drilling stage. Drilling may begin with a small number of exploratory drill holes on select targets. After this drilling stage, extensive, close-spaced drilling (called “development drilling”) is conducted. Finally, pending good results, “reserve drilling” is conducted, which is the type of drilling which makes the final assessment of the deposit before actual mining begins. Generally, some amount of drilling will continue throughout the life of the mine, as further definition is required and new information is obtained and used to refine the deposit model.
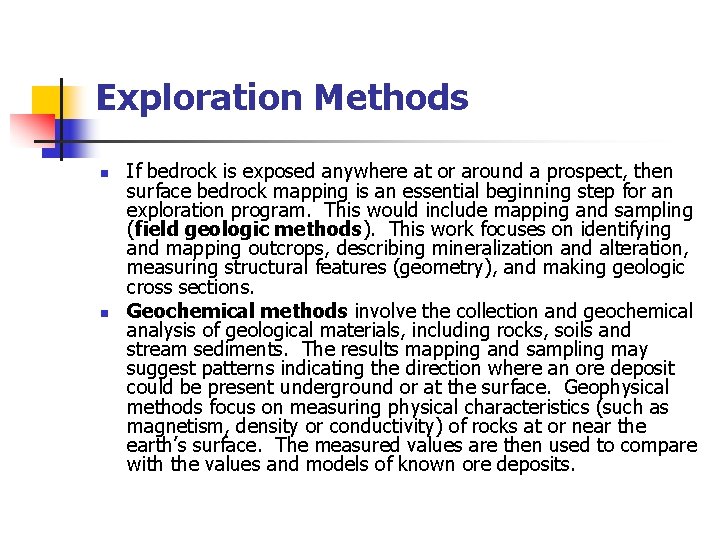
Exploration Methods n n If bedrock is exposed anywhere at or around a prospect, then surface bedrock mapping is an essential beginning step for an exploration program. This would include mapping and sampling (field geologic methods). This work focuses on identifying and mapping outcrops, describing mineralization and alteration, measuring structural features (geometry), and making geologic cross sections. Geochemical methods involve the collection and geochemical analysis of geological materials, including rocks, soils and stream sediments. The results mapping and sampling may suggest patterns indicating the direction where an ore deposit could be present underground or at the surface. Geophysical methods focus on measuring physical characteristics (such as magnetism, density or conductivity) of rocks at or near the earth’s surface. The measured values are then used to compare with the values and models of known ore deposits.
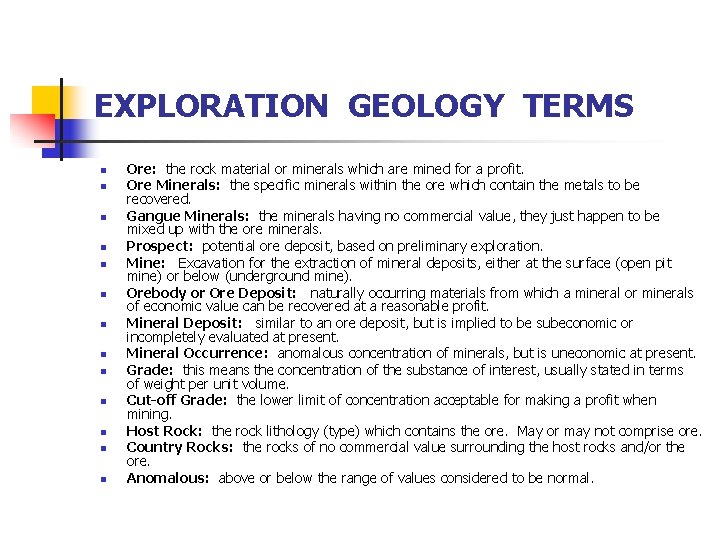
EXPLORATION GEOLOGY TERMS n n n n Ore: the rock material or minerals which are mined for a profit. Ore Minerals: the specific minerals within the ore which contain the metals to be recovered. Gangue Minerals: the minerals having no commercial value, they just happen to be mixed up with the ore minerals. Prospect: potential ore deposit, based on preliminary exploration. Mine: Excavation for the extraction of mineral deposits, either at the surface (open pit mine) or below (underground mine). Orebody or Ore Deposit: naturally occurring materials from which a mineral or minerals of economic value can be recovered at a reasonable profit. Mineral Deposit: similar to an ore deposit, but is implied to be subeconomic or incompletely evaluated at present. Mineral Occurrence: anomalous concentration of minerals, but is uneconomic at present. Grade: this means the concentration of the substance of interest, usually stated in terms of weight per unit volume. Cut-off Grade: the lower limit of concentration acceptable for making a profit when mining. Host Rock: the rock lithology (type) which contains the ore. May or may not comprise ore. Country Rocks: the rocks of no commercial value surrounding the host rocks and/or the ore. Anomalous: above or below the range of values considered to be normal.
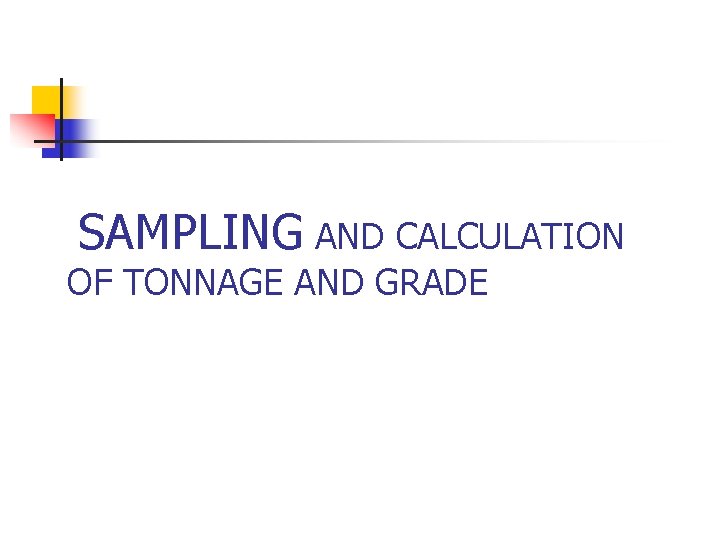
SAMPLING AND CALCULATION OF TONNAGE AND GRADE
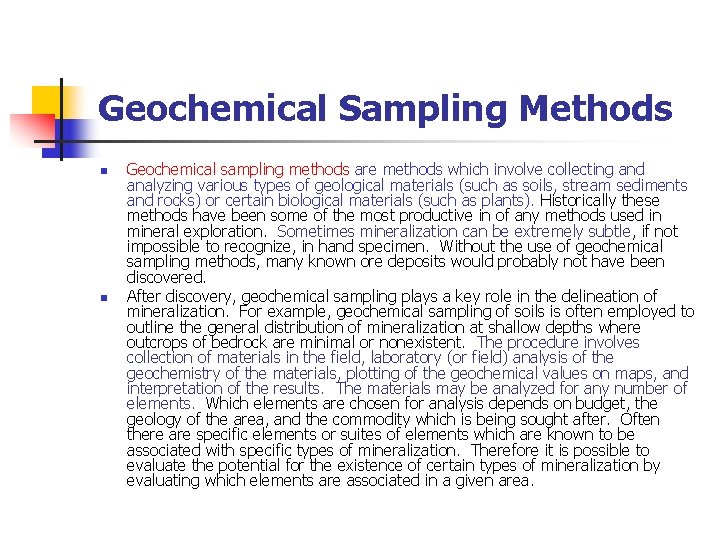
Geochemical Sampling Methods n n Geochemical sampling methods are methods which involve collecting and analyzing various types of geological materials (such as soils, stream sediments and rocks) or certain biological materials (such as plants). Historically these methods have been some of the most productive in of any methods used in mineral exploration. Sometimes mineralization can be extremely subtle, if not impossible to recognize, in hand specimen. Without the use of geochemical sampling methods, many known ore deposits would probably not have been discovered. After discovery, geochemical sampling plays a key role in the delineation of mineralization. For example, geochemical sampling of soils is often employed to outline the general distribution of mineralization at shallow depths where outcrops of bedrock are minimal or nonexistent. The procedure involves collection of materials in the field, laboratory (or field) analysis of the geochemistry of the materials, plotting of the geochemical values on maps, and interpretation of the results. The materials may be analyzed for any number of elements. Which elements are chosen for analysis depends on budget, the geology of the area, and the commodity which is being sought after. Often there are specific elements or suites of elements which are known to be associated with specific types of mineralization. Therefore it is possible to evaluate the potential for the existence of certain types of mineralization by evaluating which elements are associated in a given area.
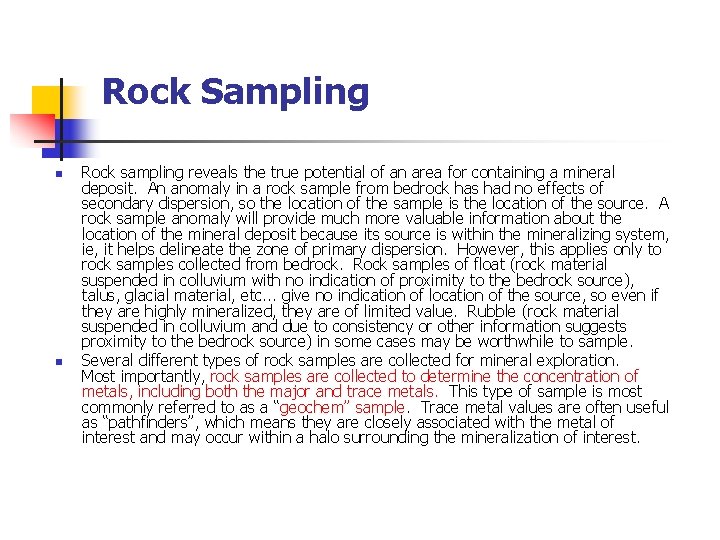
Rock Sampling n n Rock sampling reveals the true potential of an area for containing a mineral deposit. An anomaly in a rock sample from bedrock has had no effects of secondary dispersion, so the location of the sample is the location of the source. A rock sample anomaly will provide much more valuable information about the location of the mineral deposit because its source is within the mineralizing system, ie, it helps delineate the zone of primary dispersion. However, this applies only to rock samples collected from bedrock. Rock samples of float (rock material suspended in colluvium with no indication of proximity to the bedrock source), talus, glacial material, etc. . . give no indication of location of the source, so even if they are highly mineralized, they are of limited value. Rubble (rock material suspended in colluvium and due to consistency or other information suggests proximity to the bedrock source) in some cases may be worthwhile to sample. Several different types of rock samples are collected for mineral exploration. Most importantly, rock samples are collected to determine the concentration of metals, including both the major and trace metals. This type of sample is most commonly referred to as a “geochem” sample. Trace metal values are often useful as “pathfinders”, which means they are closely associated with the metal of interest and may occur within a halo surrounding the mineralization of interest.
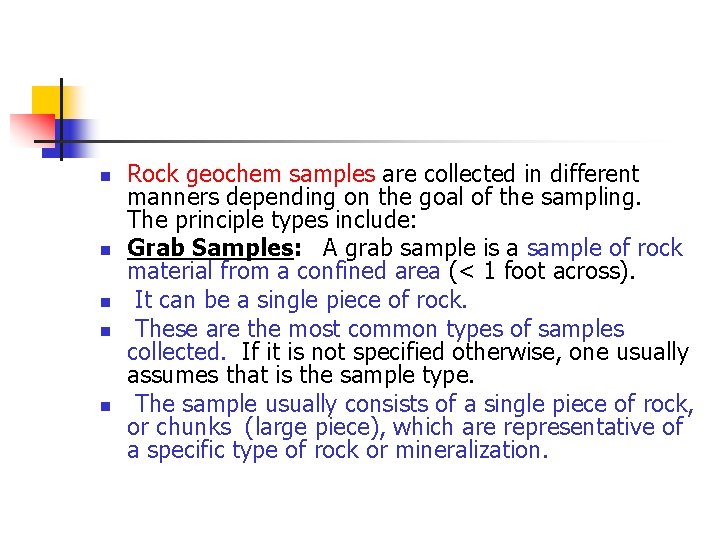
n n n Rock geochem samples are collected in different manners depending on the goal of the sampling. The principle types include: Grab Samples: A grab sample is a sample of rock material from a confined area (< 1 foot across). It can be a single piece of rock. These are the most common types of samples collected. If it is not specified otherwise, one usually assumes that is the sample type. The sample usually consists of a single piece of rock, or chunks (large piece), which are representative of a specific type of rock or mineralization.
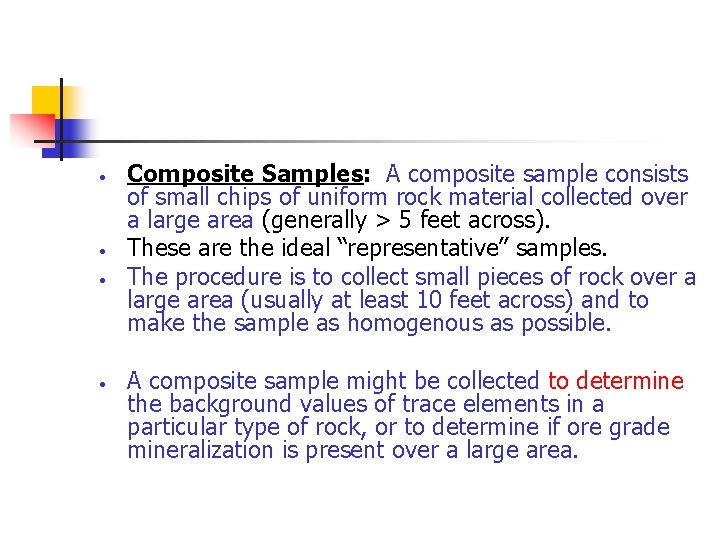
Composite Samples: A composite sample consists of small chips of uniform rock material collected over a large area (generally > 5 feet across). These are the ideal “representative” samples. The procedure is to collect small pieces of rock over a large area (usually at least 10 feet across) and to make the sample as homogenous as possible. A composite sample might be collected to determine the background values of trace elements in a particular type of rock, or to determine if ore grade mineralization is present over a large area.
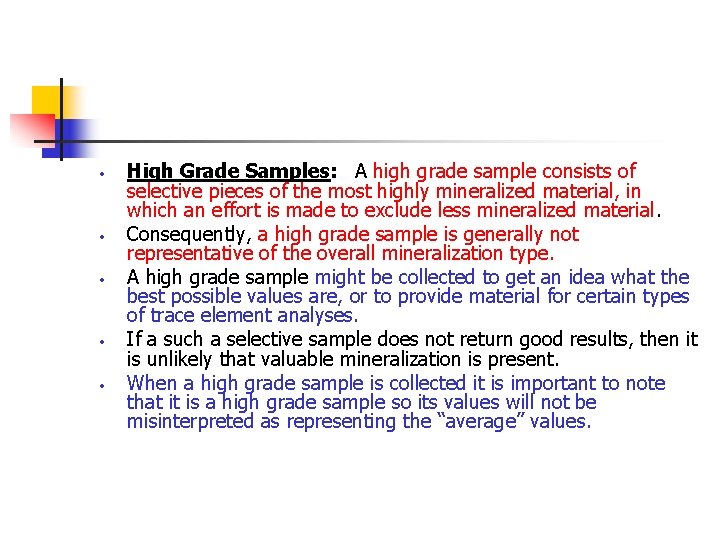
High Grade Samples: A high grade sample consists of selective pieces of the most highly mineralized material, in which an effort is made to exclude less mineralized material. Consequently, a high grade sample is generally not representative of the overall mineralization type. A high grade sample might be collected to get an idea what the best possible values are, or to provide material for certain types of trace element analyses. If a such a selective sample does not return good results, then it is unlikely that valuable mineralization is present. When a high grade sample is collected it is important to note that it is a high grade sample so its values will not be misinterpreted as representing the “average” values.
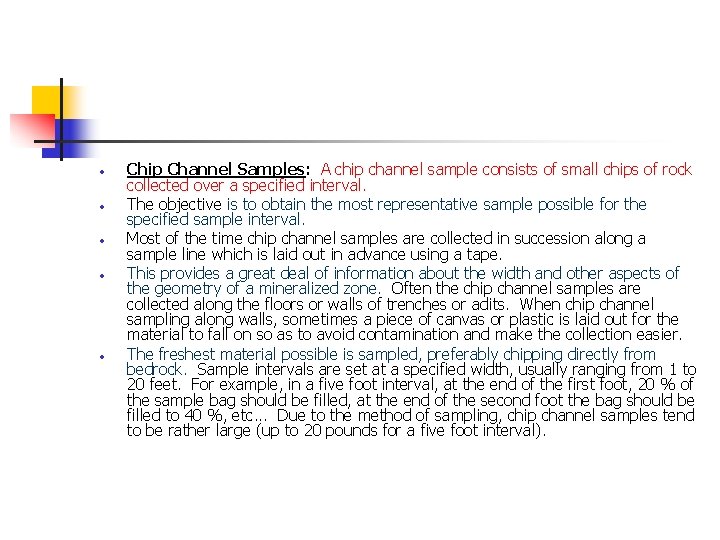
Chip Channel Samples: A chip channel sample consists of small chips of rock collected over a specified interval. The objective is to obtain the most representative sample possible for the specified sample interval. Most of the time chip channel samples are collected in succession along a sample line which is laid out in advance using a tape. This provides a great deal of information about the width and other aspects of the geometry of a mineralized zone. Often the chip channel samples are collected along the floors or walls of trenches or adits. When chip channel sampling along walls, sometimes a piece of canvas or plastic is laid out for the material to fall on so as to avoid contamination and make the collection easier. The freshest material possible is sampled, preferably chipping directly from bedrock. Sample intervals are set at a specified width, usually ranging from 1 to 20 feet. For example, in a five foot interval, at the end of the first foot, 20 % of the sample bag should be filled, at the end of the second foot the bag should be filled to 40 %, etc. . . Due to the method of sampling, chip channel samples tend to be rather large (up to 20 pounds for a five foot interval).
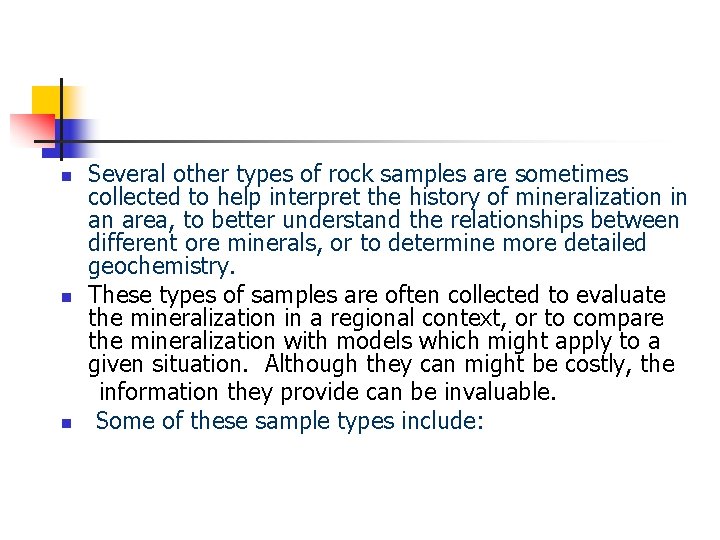
Several other types of rock samples are sometimes collected to help interpret the history of mineralization in an area, to better understand the relationships between different ore minerals, or to determine more detailed geochemistry. n These types of samples are often collected to evaluate the mineralization in a regional context, or to compare the mineralization with models which might apply to a given situation. Although they can might be costly, the information they provide can be invaluable. n Some of these sample types include: n
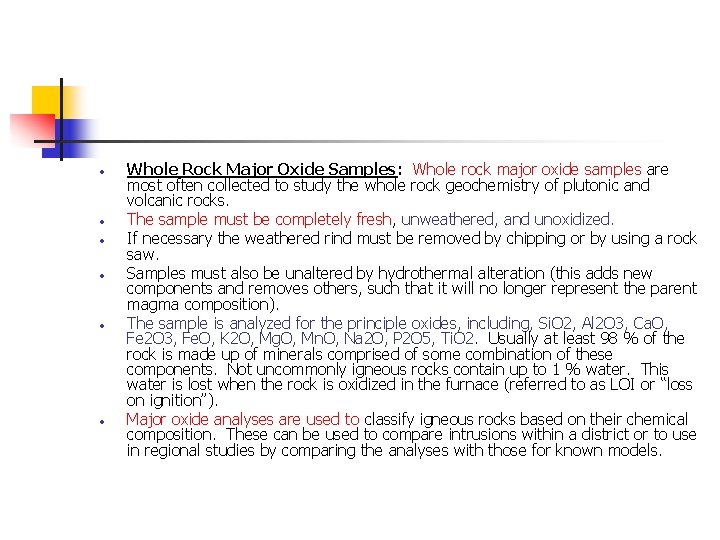
Whole Rock Major Oxide Samples: Whole rock major oxide samples are most often collected to study the whole rock geochemistry of plutonic and volcanic rocks. The sample must be completely fresh, unweathered, and unoxidized. If necessary the weathered rind must be removed by chipping or by using a rock saw. Samples must also be unaltered by hydrothermal alteration (this adds new components and removes others, such that it will no longer represent the parent magma composition). The sample is analyzed for the principle oxides, including, Si. O 2, Al 2 O 3, Ca. O, Fe 2 O 3, Fe. O, K 2 O, Mg. O, Mn. O, Na 2 O, P 2 O 5, Ti. O 2. Usually at least 98 % of the rock is made up of minerals comprised of some combination of these components. Not uncommonly igneous rocks contain up to 1 % water. This water is lost when the rock is oxidized in the furnace (referred to as LOI or “loss on ignition”). Major oxide analyses are used to classify igneous rocks based on their chemical composition. These can be used to compare intrusions within a district or to use in regional studies by comparing the analyses with those for known models.
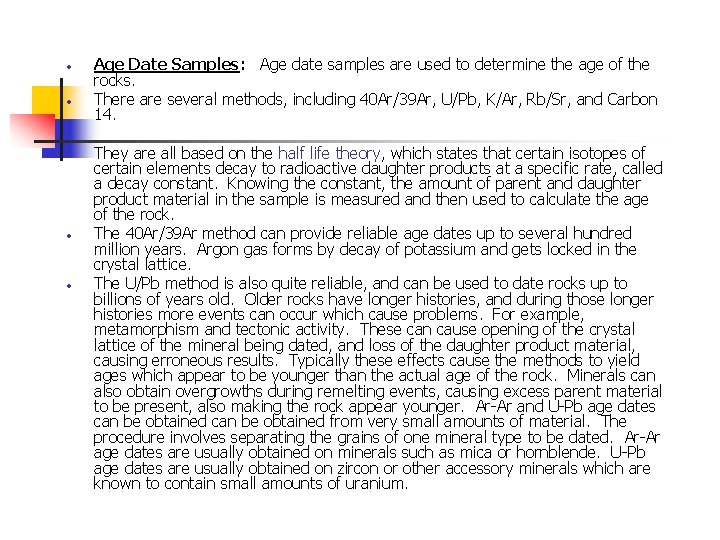
Age Date Samples: Age date samples are used to determine the age of the rocks. There are several methods, including 40 Ar/39 Ar, U/Pb, K/Ar, Rb/Sr, and Carbon 14. They are all based on the half life theory, which states that certain isotopes of certain elements decay to radioactive daughter products at a specific rate, called a decay constant. Knowing the constant, the amount of parent and daughter product material in the sample is measured and then used to calculate the age of the rock. The 40 Ar/39 Ar method can provide reliable age dates up to several hundred million years. Argon gas forms by decay of potassium and gets locked in the crystal lattice. The U/Pb method is also quite reliable, and can be used to date rocks up to billions of years old. Older rocks have longer histories, and during those longer histories more events can occur which cause problems. For example, metamorphism and tectonic activity. These can cause opening of the crystal lattice of the mineral being dated, and loss of the daughter product material, causing erroneous results. Typically these effects cause the methods to yield ages which appear to be younger than the actual age of the rock. Minerals can also obtain overgrowths during remelting events, causing excess parent material to be present, also making the rock appear younger. Ar-Ar and U-Pb age dates can be obtained from very small amounts of material. The procedure involves separating the grains of one mineral type to be dated. Ar-Ar age dates are usually obtained on minerals such as mica or hornblende. U-Pb age dates are usually obtained on zircon or other accessory minerals which are known to contain small amounts of uranium.
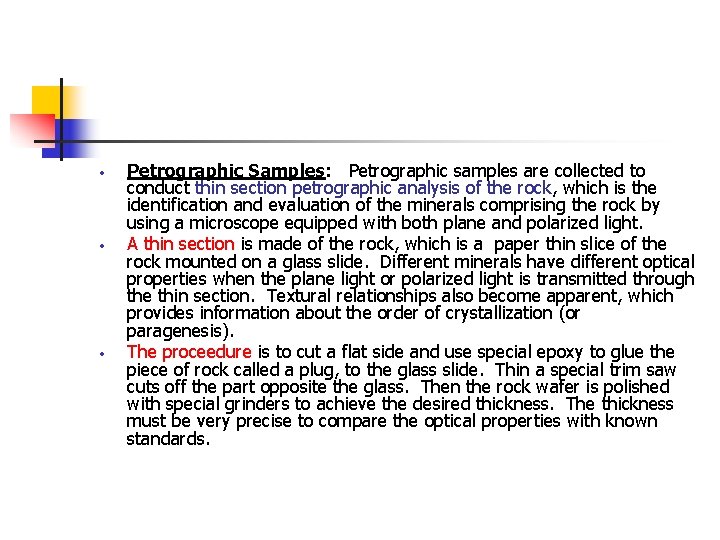
Petrographic Samples: Petrographic samples are collected to conduct thin section petrographic analysis of the rock, which is the identification and evaluation of the minerals comprising the rock by using a microscope equipped with both plane and polarized light. A thin section is made of the rock, which is a paper thin slice of the rock mounted on a glass slide. Different minerals have different optical properties when the plane light or polarized light is transmitted through the thin section. Textural relationships also become apparent, which provides information about the order of crystallization (or paragenesis). The proceedure is to cut a flat side and use special epoxy to glue the piece of rock called a plug, to the glass slide. Thin a special trim saw cuts off the part opposite the glass. Then the rock wafer is polished with special grinders to achieve the desired thickness. The thickness must be very precise to compare the optical properties with known standards.
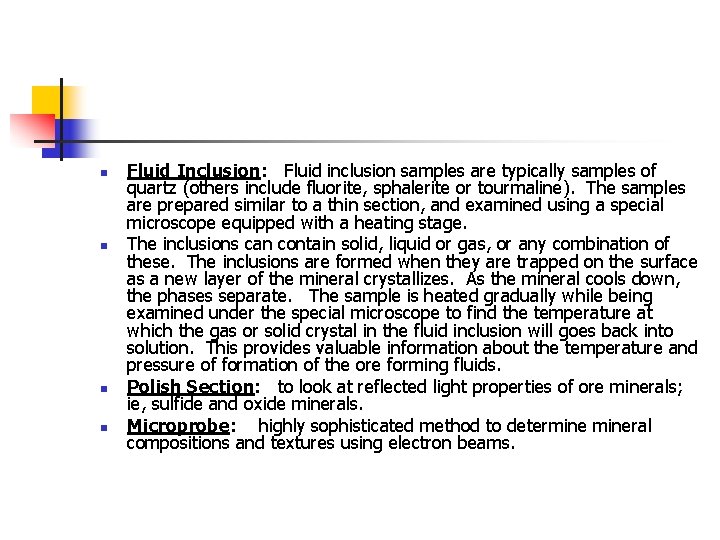
n n Fluid Inclusion: Fluid inclusion samples are typically samples of quartz (others include fluorite, sphalerite or tourmaline). The samples are prepared similar to a thin section, and examined using a special microscope equipped with a heating stage. The inclusions can contain solid, liquid or gas, or any combination of these. The inclusions are formed when they are trapped on the surface as a new layer of the mineral crystallizes. As the mineral cools down, the phases separate. The sample is heated gradually while being examined under the special microscope to find the temperature at which the gas or solid crystal in the fluid inclusion will goes back into solution. This provides valuable information about the temperature and pressure of formation of the ore forming fluids. Polish Section: to look at reflected light properties of ore minerals; ie, sulfide and oxide minerals. Microprobe: highly sophisticated method to determineral compositions and textures using electron beams.
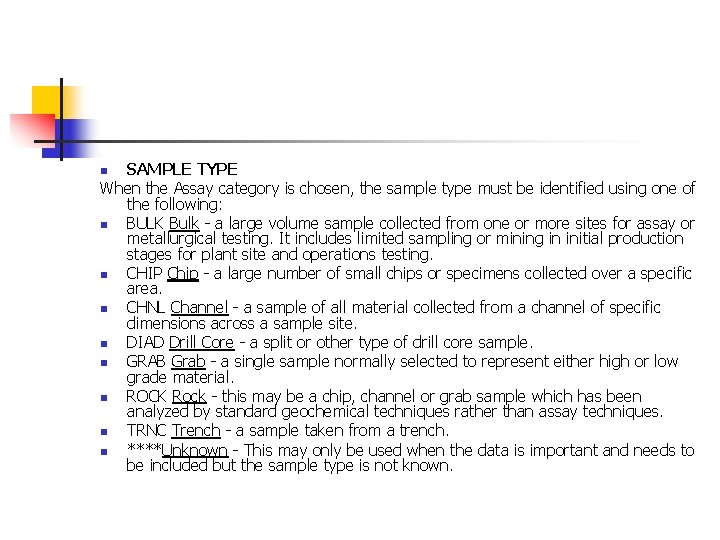
SAMPLE TYPE When the Assay category is chosen, the sample type must be identified using one of the following: n BULK Bulk - a large volume sample collected from one or more sites for assay or metallurgical testing. It includes limited sampling or mining in initial production stages for plant site and operations testing. n CHIP Chip - a large number of small chips or specimens collected over a specific area. n CHNL Channel - a sample of all material collected from a channel of specific dimensions across a sample site. n DIAD Drill Core - a split or other type of drill core sample. n GRAB Grab - a single sample normally selected to represent either high or low grade material. n ROCK Rock - this may be a chip, channel or grab sample which has been analyzed by standard geochemical techniques rather than assay techniques. n TRNC Trench - a sample taken from a trench. n ****Unknown - This may only be used when the data is important and needs to be included but the sample type is not known. n
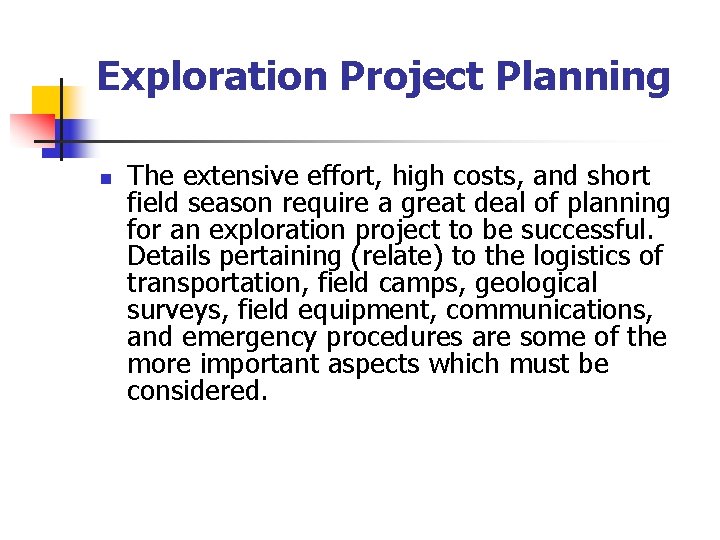
Exploration Project Planning n The extensive effort, high costs, and short field season require a great deal of planning for an exploration project to be successful. Details pertaining (relate) to the logistics of transportation, field camps, geological surveys, field equipment, communications, and emergency procedures are some of the more important aspects which must be considered.
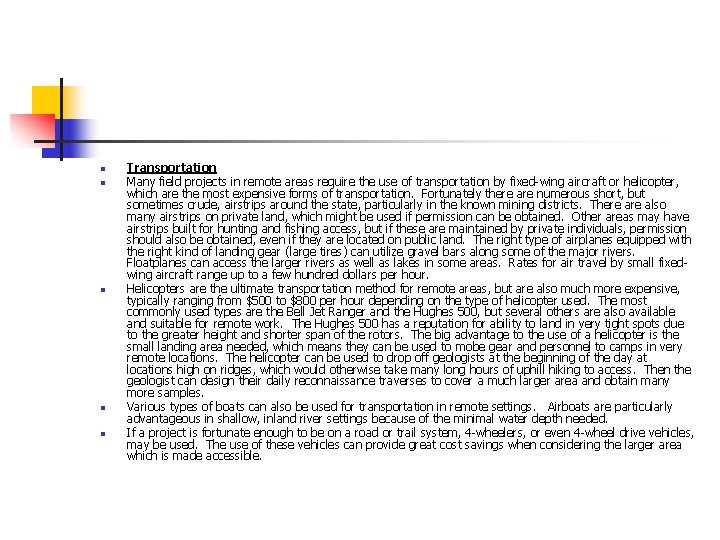
n n n Transportation Many field projects in remote areas require the use of transportation by fixed-wing aircraft or helicopter, which are the most expensive forms of transportation. Fortunately there are numerous short, but sometimes crude, airstrips around the state, particularly in the known mining districts. There also many airstrips on private land, which might be used if permission can be obtained. Other areas may have airstrips built for hunting and fishing access, but if these are maintained by private individuals, permission should also be obtained, even if they are located on public land. The right type of airplanes equipped with the right kind of landing gear (large tires) can utilize gravel bars along some of the major rivers. Floatplanes can access the larger rivers as well as lakes in some areas. Rates for air travel by small fixedwing aircraft range up to a few hundred dollars per hour. Helicopters are the ultimate transportation method for remote areas, but are also much more expensive, typically ranging from $500 to $800 per hour depending on the type of helicopter used. The most commonly used types are the Bell Jet Ranger and the Hughes 500, but several others are also available and suitable for remote work. The Hughes 500 has a reputation for ability to land in very tight spots due to the greater height and shorter span of the rotors. The big advantage to the use of a helicopter is the small landing area needed, which means they can be used to mobe gear and personnel to camps in very remote locations. The helicopter can be used to drop off geologists at the beginning of the day at locations high on ridges, which would otherwise take many long hours of uphill hiking to access. Then the geologist can design their daily reconnaissance traverses to cover a much larger area and obtain many more samples. Various types of boats can also be used for transportation in remote settings. Airboats are particularly advantageous in shallow, inland river settings because of the minimal water depth needed. If a project is fortunate enough to be on a road or trail system, 4 -wheelers, or even 4 -wheel drive vehicles, may be used. The use of these vehicles can provide great cost savings when considering the larger area which is made accessible.
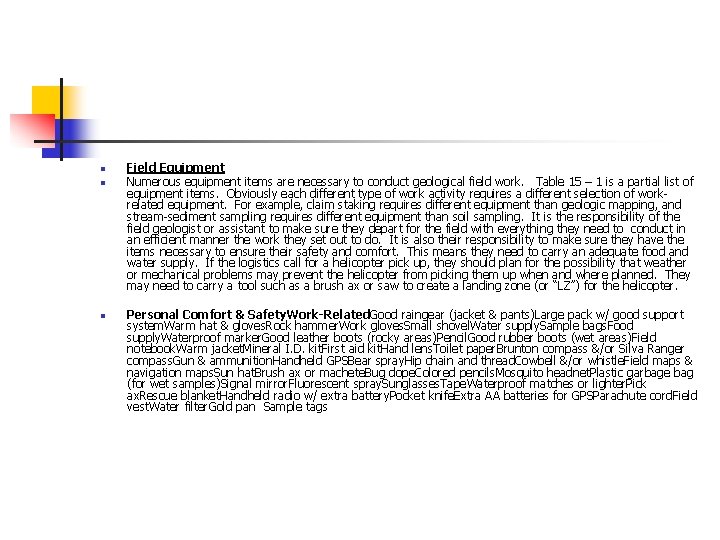
n n n Field Equipment Numerous equipment items are necessary to conduct geological field work. Table 15 – 1 is a partial list of equipment items. Obviously each different type of work activity requires a different selection of workrelated equipment. For example, claim staking requires different equipment than geologic mapping, and stream-sediment sampling requires different equipment than soil sampling. It is the responsibility of the field geologist or assistant to make sure they depart for the field with everything they need to conduct in an efficient manner the work they set out to do. It is also their responsibility to make sure they have the items necessary to ensure their safety and comfort. This means they need to carry an adequate food and water supply. If the logistics call for a helicopter pick up, they should plan for the possibility that weather or mechanical problems may prevent the helicopter from picking them up when and where planned. They may need to carry a tool such as a brush ax or saw to create a landing zone (or “LZ”) for the helicopter. Personal Comfort & Safety. Work-Related. Good raingear (jacket & pants)Large pack w/ good support system. Warm hat & gloves. Rock hammer. Work gloves. Small shovel. Water supply. Sample bags. Food supply. Waterproof marker. Good leather boots (rocky areas)Pencil. Good rubber boots (wet areas)Field notebook. Warm jacket. Mineral I. D. kit. First aid kit. Hand lens. Toilet paper. Brunton compass &/or Silva Ranger compass. Gun & ammunition. Handheld GPSBear spray. Hip chain and thread. Cowbell &/or whistle. Field maps & navigation maps. Sun hat. Brush ax or machete. Bug dope. Colored pencils. Mosquito headnet. Plastic garbage bag (for wet samples)Signal mirror. Fluorescent spray. Sunglasses. Tape. Waterproof matches or lighter. Pick ax. Rescue blanket. Handheld radio w/ extra battery. Pocket knife. Extra AA batteries for GPSParachute cord. Field vest. Water filter. Gold pan Sample tags
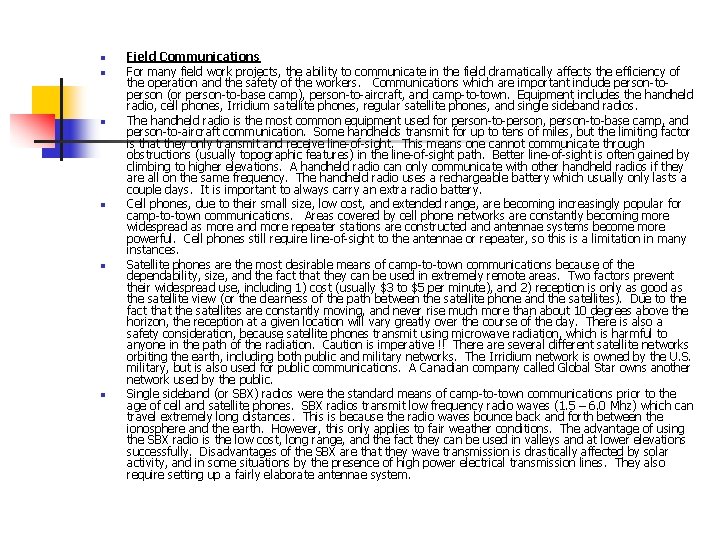
n n n Field Communications For many field work projects, the ability to communicate in the field dramatically affects the efficiency of the operation and the safety of the workers. Communications which are important include person-toperson (or person-to-base camp), person-to-aircraft, and camp-to-town. Equipment includes the handheld radio, cell phones, Irridium satellite phones, regular satellite phones, and single sideband radios. The handheld radio is the most common equipment used for person-to-person, person-to-base camp, and person-to-aircraft communication. Some handhelds transmit for up to tens of miles, but the limiting factor is that they only transmit and receive line-of-sight. This means one cannot communicate through obstructions (usually topographic features) in the line-of-sight path. Better line-of-sight is often gained by climbing to higher elevations. A handheld radio can only communicate with other handheld radios if they are all on the same frequency. The handheld radio uses a rechargeable battery which usually only lasts a couple days. It is important to always carry an extra radio battery. Cell phones, due to their small size, low cost, and extended range, are becoming increasingly popular for camp-to-town communications. Areas covered by cell phone networks are constantly becoming more widespread as more and more repeater stations are constructed antennae systems become more powerful. Cell phones still require line-of-sight to the antennae or repeater, so this is a limitation in many instances. Satellite phones are the most desirable means of camp-to-town communications because of the dependability, size, and the fact that they can be used in extremely remote areas. Two factors prevent their widespread use, including 1) cost (usually $3 to $5 per minute), and 2) reception is only as good as the satellite view (or the clearness of the path between the satellite phone and the satellites). Due to the fact that the satellites are constantly moving, and never rise much more than about 10 degrees above the horizon, the reception at a given location will vary greatly over the course of the day. There is also a safety consideration, because satellite phones transmit using microwave radiation, which is harmful to anyone in the path of the radiation. Caution is imperative !! There are several different satellite networks orbiting the earth, including both public and military networks. The Irridium network is owned by the U. S. military, but is also used for public communications. A Canadian company called Global Star owns another network used by the public. Single sideband (or SBX) radios were the standard means of camp-to-town communications prior to the age of cell and satellite phones. SBX radios transmit low frequency radio waves (1. 5 – 6. 0 Mhz) which can travel extremely long distances. This is because the radio waves bounce back and forth between the ionosphere and the earth. However, this only applies to fair weather conditions. The advantage of using the SBX radio is the low cost, long range, and the fact they can be used in valleys and at lower elevations successfully. Disadvantages of the SBX are that they wave transmission is drastically affected by solar activity, and in some situations by the presence of high power electrical transmission lines. They also require setting up a fairly elaborate antennae system.
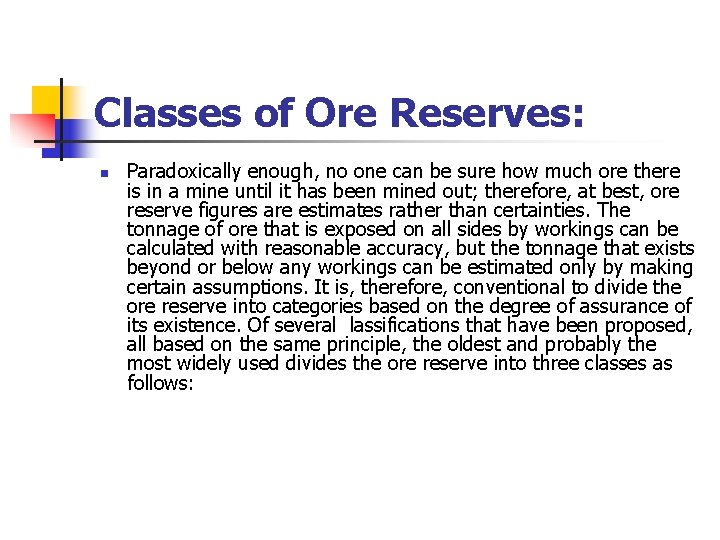
Classes of Ore Reserves: n Paradoxically enough, no one can be sure how much ore there is in a mine until it has been mined out; therefore, at best, ore reserve figures are estimates rather than certainties. The tonnage of ore that is exposed on all sides by workings can be calculated with reasonable accuracy, but the tonnage that exists beyond or below any workings can be estimated only by making certain assumptions. It is, therefore, conventional to divide the ore reserve into categories based on the degree of assurance of its existence. Of several lassifications that have been proposed, all based on the same principle, the oldest and probably the most widely used divides the ore reserve into three classes as follows:
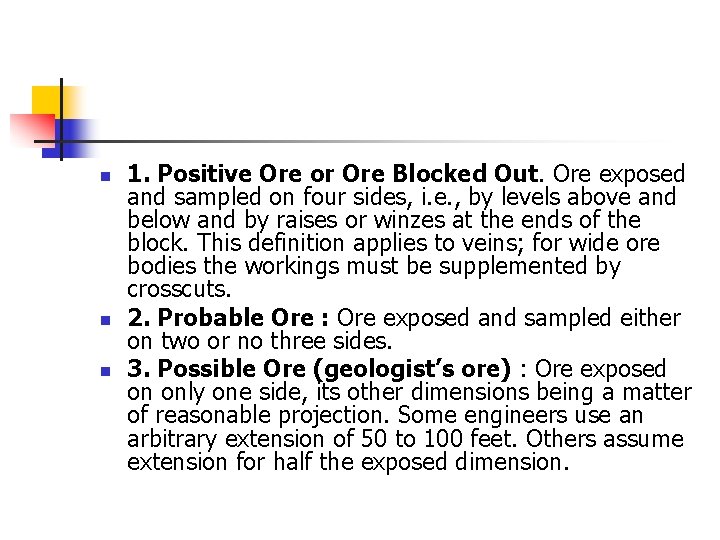
n n n 1. Positive Ore or Ore Blocked Out. Ore exposed and sampled on four sides, i. e. , by levels above and below and by raises or winzes at the ends of the block. This definition applies to veins; for wide ore bodies the workings must be supplemented by crosscuts. 2. Probable Ore : Ore exposed and sampled either on two or no three sides. 3. Possible Ore (geologist’s ore) : Ore exposed on only one side, its other dimensions being a matter of reasonable projection. Some engineers use an arbitrary extension of 50 to 100 feet. Others assume extension for half the exposed dimension.
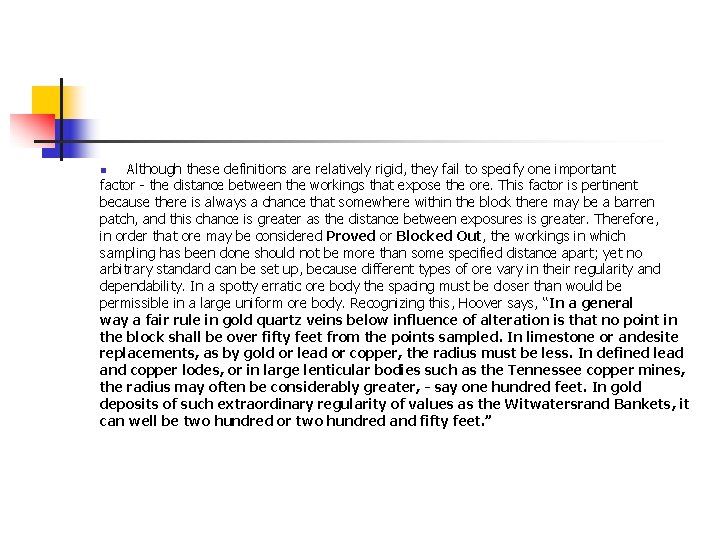
Although these definitions are relatively rigid, they fail to specify one important factor - the distance between the workings that expose the ore. This factor is pertinent because there is always a chance that somewhere within the block there may be a barren patch, and this chance is greater as the distance between exposures is greater. Therefore, in order that ore may be considered Proved or Blocked Out, the workings in which sampling has been done should not be more than some specified distance apart; yet no arbitrary standard can be set up, because different types of ore vary in their regularity and dependability. In a spotty erratic ore body the spacing must be closer than would be permissible in a large uniform ore body. Recognizing this, Hoover says, “In a general way a fair rule in gold quartz veins below influence of alteration is that no point in the block shall be over fifty feet from the points sampled. In limestone or andesite replacements, as by gold or lead or copper, the radius must be less. In defined lead and copper lodes, or in large lenticular bodies such as the Tennessee copper mines, the radius may often be considerably greater, - say one hundred feet. In gold deposits of such extraordinary regularity of values as the Witwatersrand Bankets, it can well be two hundred or two hundred and fifty feet. ” n
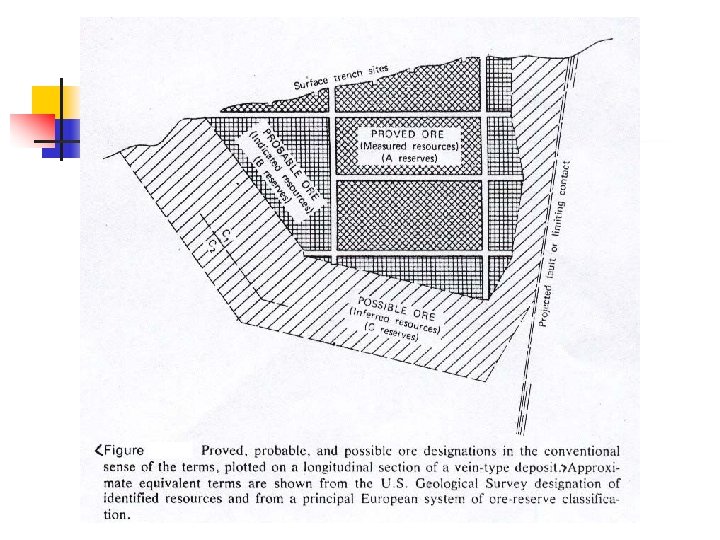
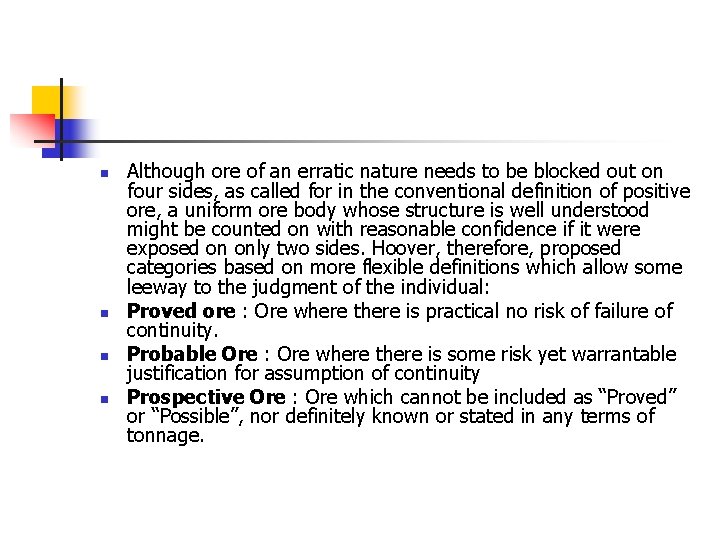
n n Although ore of an erratic nature needs to be blocked out on four sides, as called for in the conventional definition of positive ore, a uniform ore body whose structure is well understood might be counted on with reasonable confidence if it were exposed on only two sides. Hoover, therefore, proposed categories based on more flexible definitions which allow some leeway to the judgment of the individual: Proved ore : Ore where there is practical no risk of failure of continuity. Probable Ore : Ore where there is some risk yet warrantable justification for assumption of continuity Prospective Ore : Ore which cannot be included as “Proved” or “Possible”, nor definitely known or stated in any terms of tonnage.
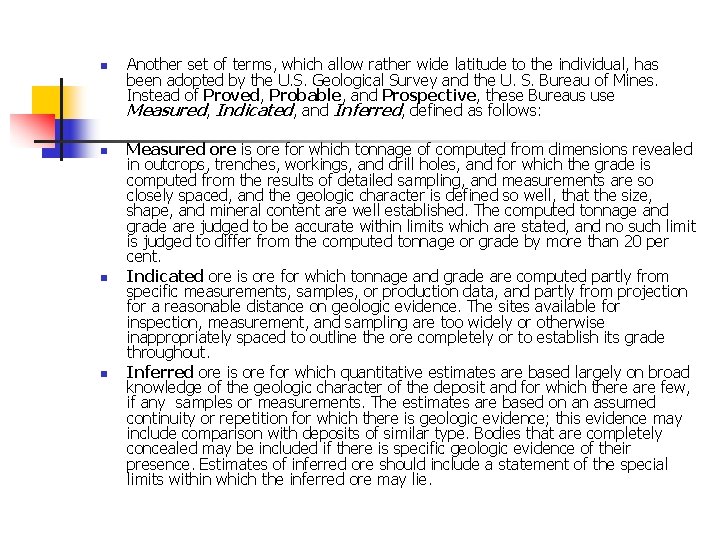
n n Another set of terms, which allow rather wide latitude to the individual, has been adopted by the U. S. Geological Survey and the U. S. Bureau of Mines. Instead of Proved, Probable, and Prospective, these Bureaus use Measured, Indicated, and Inferred, defined as follows: Measured ore is ore for which tonnage of computed from dimensions revealed in outcrops, trenches, workings, and drill holes, and for which the grade is computed from the results of detailed sampling, and measurements are so closely spaced, and the geologic character is defined so well, that the size, shape, and mineral content are well established. The computed tonnage and grade are judged to be accurate within limits which are stated, and no such limit is judged to differ from the computed tonnage or grade by more than 20 per cent. Indicated ore is ore for which tonnage and grade are computed partly from specific measurements, samples, or production data, and partly from projection for a reasonable distance on geologic evidence. The sites available for inspection, measurement, and sampling are too widely or otherwise inappropriately spaced to outline the ore completely or to establish its grade throughout. Inferred ore is ore for which quantitative estimates are based largely on broad knowledge of the geologic character of the deposit and for which there are few, if any samples or measurements. The estimates are based on an assumed continuity or repetition for which there is geologic evidence; this evidence may include comparison with deposits of similar type. Bodies that are completely concealed may be included if there is specific geologic evidence of their presence. Estimates of inferred ore should include a statement of the special limits within which the inferred ore may lie.
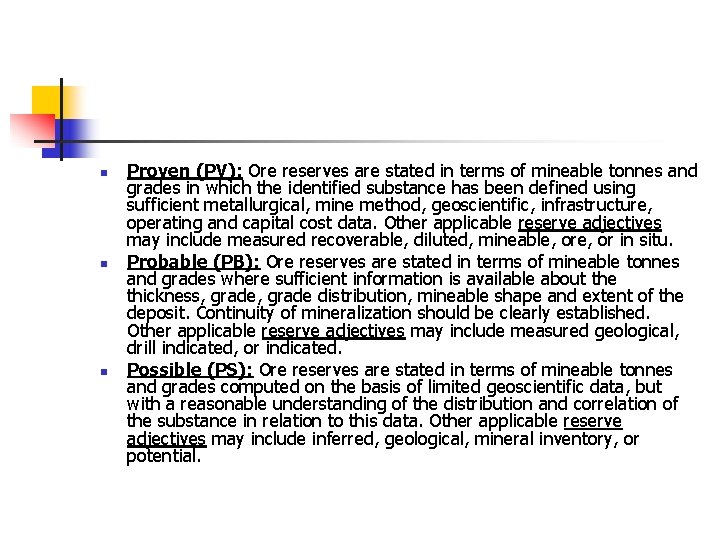
n n n Proven (PV): Ore reserves are stated in terms of mineable tonnes and grades in which the identified substance has been defined using sufficient metallurgical, mine method, geoscientific, infrastructure, operating and capital cost data. Other applicable reserve adjectives may include measured recoverable, diluted, mineable, or in situ. Probable (PB): Ore reserves are stated in terms of mineable tonnes and grades where sufficient information is available about the thickness, grade distribution, mineable shape and extent of the deposit. Continuity of mineralization should be clearly established. Other applicable reserve adjectives may include measured geological, drill indicated, or indicated. Possible (PS): Ore reserves are stated in terms of mineable tonnes and grades computed on the basis of limited geoscientific data, but with a reasonable understanding of the distribution and correlation of the substance in relation to this data. Other applicable reserve adjectives may include inferred, geological, mineral inventory, or potential.
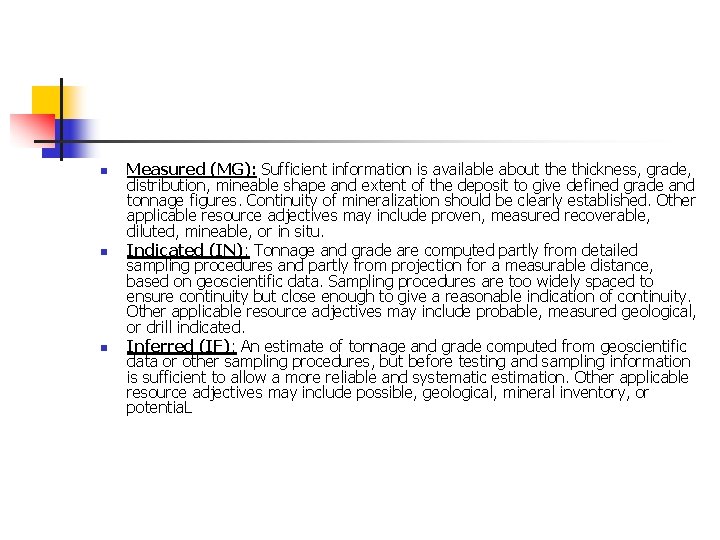
n n n Measured (MG): Sufficient information is available about the thickness, grade, distribution, mineable shape and extent of the deposit to give defined grade and tonnage figures. Continuity of mineralization should be clearly established. Other applicable resource adjectives may include proven, measured recoverable, diluted, mineable, or in situ. Indicated (IN): Tonnage and grade are computed partly from detailed sampling procedures and partly from projection for a measurable distance, based on geoscientific data. Sampling procedures are too widely spaced to ensure continuity but close enough to give a reasonable indication of continuity. Other applicable resource adjectives may include probable, measured geological, or drill indicated. Inferred (IF): An estimate of tonnage and grade computed from geoscientific data or other sampling procedures, but before testing and sampling information is sufficient to allow a more reliable and systematic estimation. Other applicable resource adjectives may include possible, geological, mineral inventory, or potentia. L
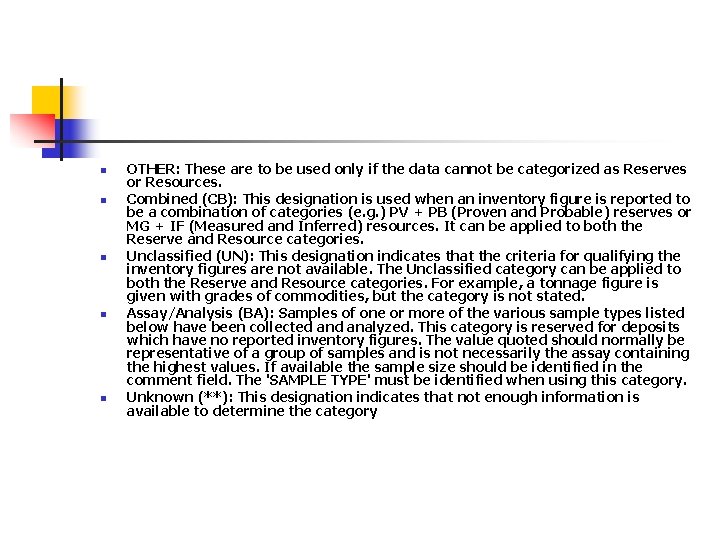
n n n OTHER: These are to be used only if the data cannot be categorized as Reserves or Resources. Combined (CB): This designation is used when an inventory figure is reported to be a combination of categories (e. g. ) PV + PB (Proven and Probable) reserves or MG + IF (Measured and Inferred) resources. It can be applied to both the Reserve and Resource categories. Unclassified (UN): This designation indicates that the criteria for qualifying the inventory figures are not available. The Unclassified category can be applied to both the Reserve and Resource categories. For example, a tonnage figure is given with grades of commodities, but the category is not stated. Assay/Analysis (BA): Samples of one or more of the various sample types listed below have been collected analyzed. This category is reserved for deposits which have no reported inventory figures. The value quoted should normally be representative of a group of samples and is not necessarily the assay containing the highest values. If available the sample size should be identified in the comment field. The 'SAMPLE TYPE' must be identified when using this category. Unknown (**): This designation indicates that not enough information is available to determine the category
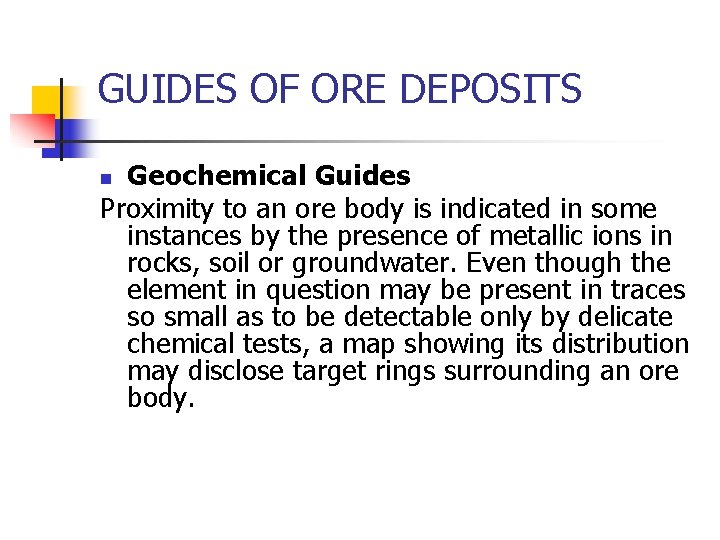
GUIDES OF ORE DEPOSITS Geochemical Guides Proximity to an ore body is indicated in some instances by the presence of metallic ions in rocks, soil or groundwater. Even though the element in question may be present in traces so small as to be detectable only by delicate chemical tests, a map showing its distribution may disclose target rings surrounding an ore body. n
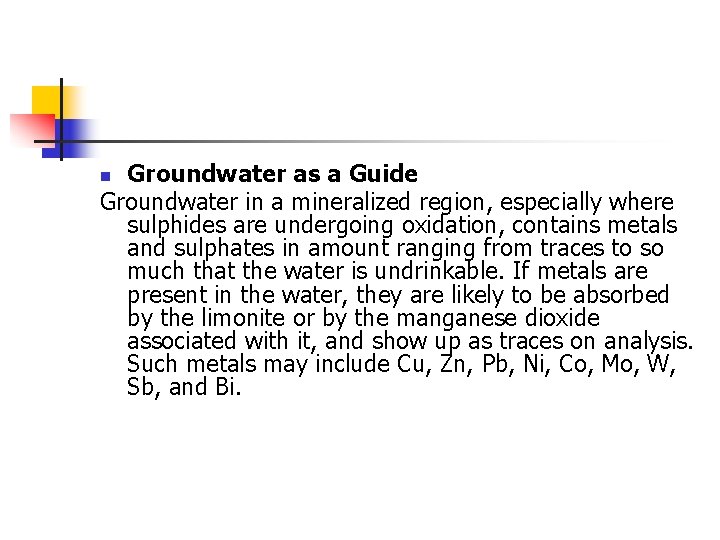
Groundwater as a Guide Groundwater in a mineralized region, especially where sulphides are undergoing oxidation, contains metals and sulphates in amount ranging from traces to so much that the water is undrinkable. If metals are present in the water, they are likely to be absorbed by the limonite or by the manganese dioxide associated with it, and show up as traces on analysis. Such metals may include Cu, Zn, Pb, Ni, Co, Mo, W, Sb, and Bi. n
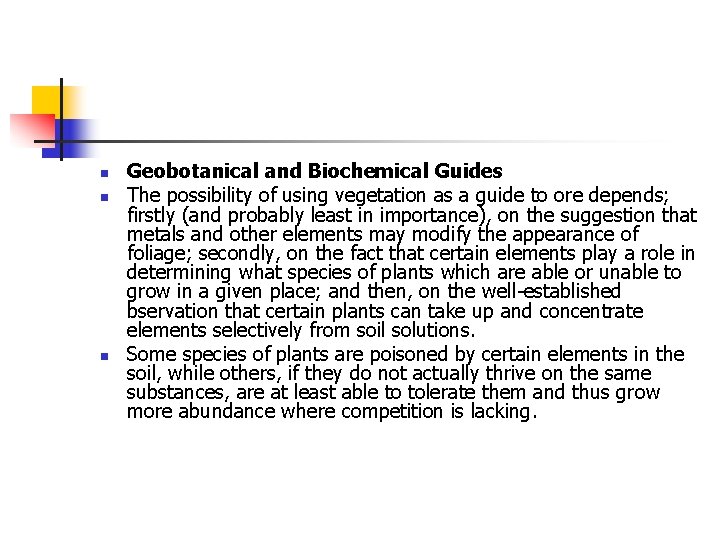
n n n Geobotanical and Biochemical Guides The possibility of using vegetation as a guide to ore depends; firstly (and probably least in importance), on the suggestion that metals and other elements may modify the appearance of foliage; secondly, on the fact that certain elements play a role in determining what species of plants which are able or unable to grow in a given place; and then, on the well-established bservation that certain plants can take up and concentrate elements selectively from soil solutions. Some species of plants are poisoned by certain elements in the soil, while others, if they do not actually thrive on the same substances, are at least able to tolerate them and thus grow more abundance where competition is lacking.
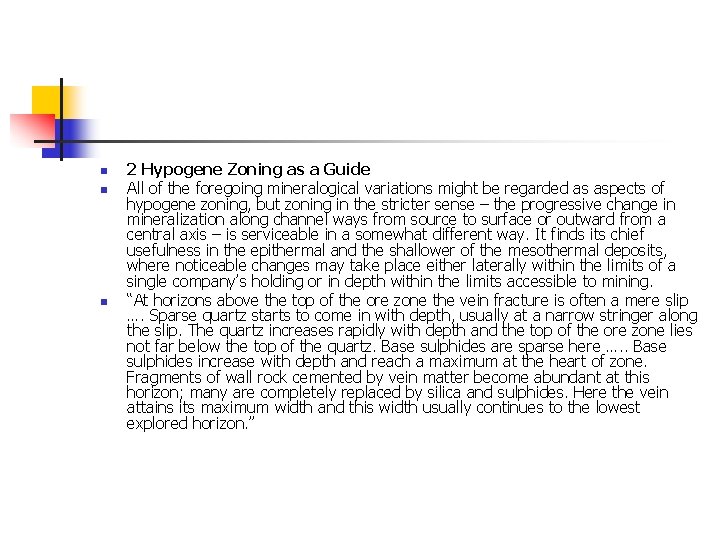
n n n 2 Hypogene Zoning as a Guide All of the foregoing mineralogical variations might be regarded as aspects of hypogene zoning, but zoning in the stricter sense – the progressive change in mineralization along channel ways from source to surface or outward from a central axis – is serviceable in a somewhat different way. It finds its chief usefulness in the epithermal and the shallower of the mesothermal deposits, where noticeable changes may take place either laterally within the limits of a single company’s holding or in depth within the limits accessible to mining. “At horizons above the top of the ore zone the vein fracture is often a mere slip …. Sparse quartz starts to come in with depth, usually at a narrow stringer along the slip. The quartz increases rapidly with depth and the top of the ore zone lies not far below the top of the quartz. Base sulphides are sparse here …. . Base sulphides increase with depth and reach a maximum at the heart of zone. Fragments of wall rock cemented by vein matter become abundant at this horizon; many are completely replaced by silica and sulphides. Here the vein attains its maximum width and this width usually continues to the lowest explored horizon. ”
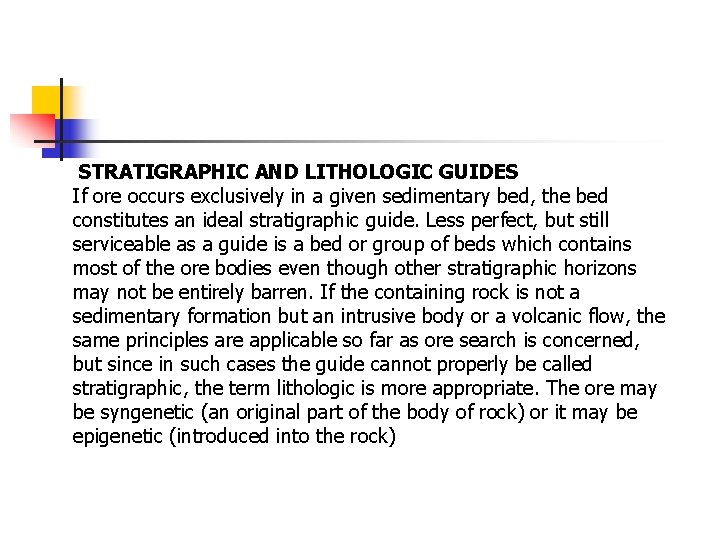
STRATIGRAPHIC AND LITHOLOGIC GUIDES If ore occurs exclusively in a given sedimentary bed, the bed constitutes an ideal stratigraphic guide. Less perfect, but still serviceable as a guide is a bed or group of beds which contains most of the ore bodies even though other stratigraphic horizons may not be entirely barren. If the containing rock is not a sedimentary formation but an intrusive body or a volcanic flow, the same principles are applicable so far as ore search is concerned, but since in such cases the guide cannot properly be called stratigraphic, the term lithologic is more appropriate. The ore may be syngenetic (an original part of the body of rock) or it may be epigenetic (introduced into the rock)
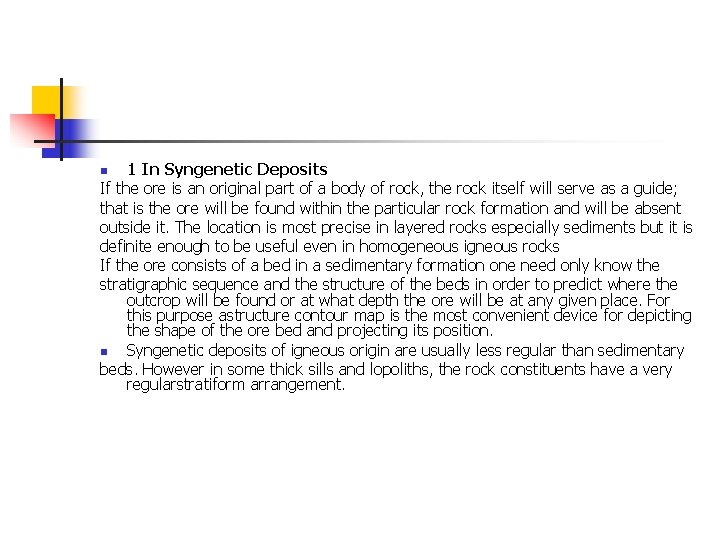
1 In Syngenetic Deposits If the ore is an original part of a body of rock, the rock itself will serve as a guide; that is the ore will be found within the particular rock formation and will be absent outside it. The location is most precise in layered rocks especially sediments but it is definite enough to be useful even in homogeneous igneous rocks If the ore consists of a bed in a sedimentary formation one need only know the stratigraphic sequence and the structure of the beds in order to predict where the outcrop will be found or at what depth the ore will be at any given place. For this purpose astructure contour map is the most convenient device for depicting the shape of the ore bed and projecting its position. n Syngenetic deposits of igneous origin are usually less regular than sedimentary beds. However in some thick sills and lopoliths, the rock constituents have a very regularstratiform arrangement. n
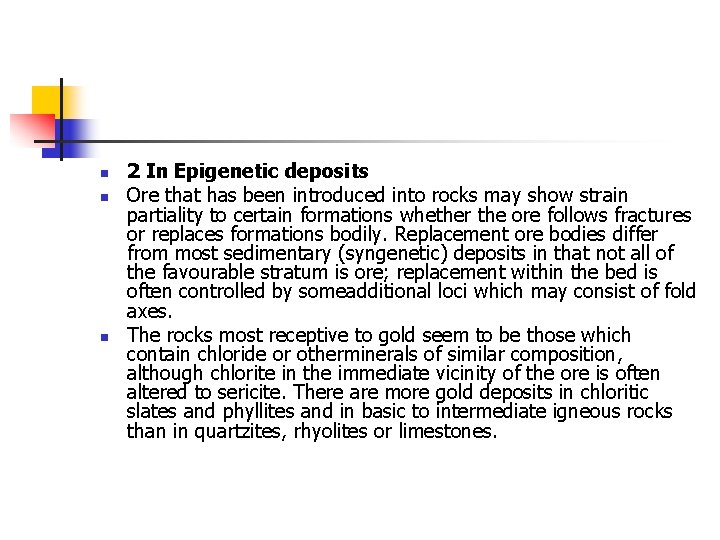
n n n 2 In Epigenetic deposits Ore that has been introduced into rocks may show strain partiality to certain formations whether the ore follows fractures or replaces formations bodily. Replacement ore bodies differ from most sedimentary (syngenetic) deposits in that not all of the favourable stratum is ore; replacement within the bed is often controlled by someadditional loci which may consist of fold axes. The rocks most receptive to gold seem to be those which contain chloride or otherminerals of similar composition, although chlorite in the immediate vicinity of the ore is often altered to sericite. There are more gold deposits in chloritic slates and phyllites and in basic to intermediate igneous rocks than in quartzites, rhyolites or limestones.
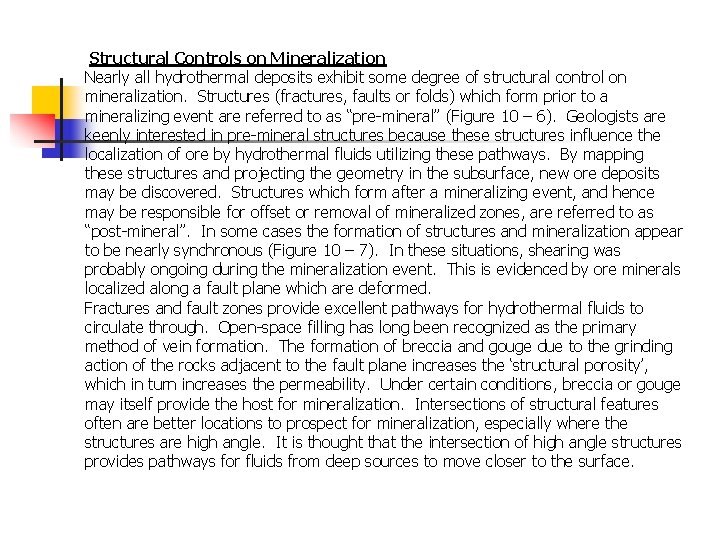
Structural Controls on Mineralization Nearly all hydrothermal deposits exhibit some degree of structural control on mineralization. Structures (fractures, faults or folds) which form prior to a mineralizing event are referred to as “pre-mineral” (Figure 10 – 6). Geologists are keenly interested in pre-mineral structures because these structures influence the localization of ore by hydrothermal fluids utilizing these pathways. By mapping these structures and projecting the geometry in the subsurface, new ore deposits may be discovered. Structures which form after a mineralizing event, and hence may be responsible for offset or removal of mineralized zones, are referred to as “post-mineral”. In some cases the formation of structures and mineralization appear to be nearly synchronous (Figure 10 – 7). In these situations, shearing was probably ongoing during the mineralization event. This is evidenced by ore minerals localized along a fault plane which are deformed. Fractures and fault zones provide excellent pathways for hydrothermal fluids to circulate through. Open-space filling has long been recognized as the primary method of vein formation. The formation of breccia and gouge due to the grinding action of the rocks adjacent to the fault plane increases the ‘structural porosity’, which in turn increases the permeability. Under certain conditions, breccia or gouge may itself provide the host for mineralization. Intersections of structural features often are better locations to prospect for mineralization, especially where the structures are high angle. It is thought that the intersection of high angle structures provides pathways for fluids from deep sources to move closer to the surface.
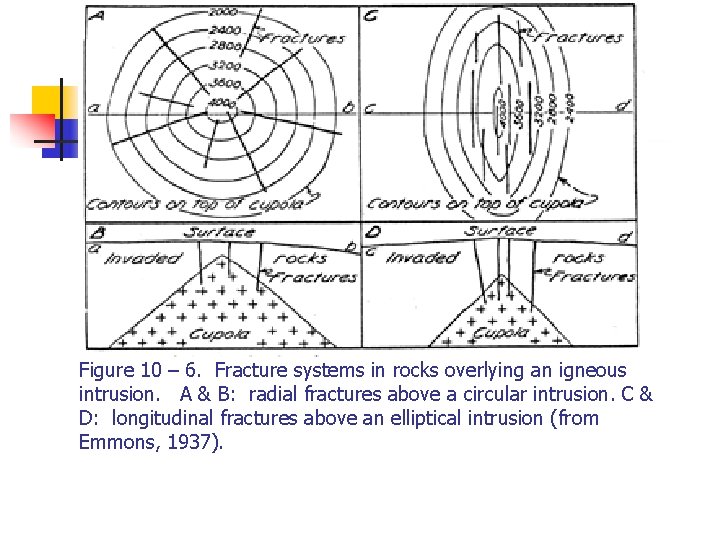
Figure 10 – 6. Fracture systems in rocks overlying an igneous intrusion. A & B: radial fractures above a circular intrusion. C & D: longitudinal fractures above an elliptical intrusion (from Emmons, 1937).
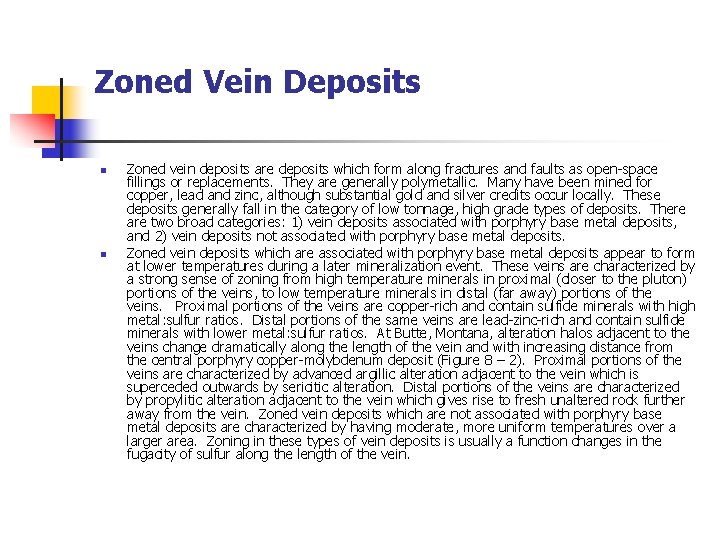
Zoned Vein Deposits n n Zoned vein deposits are deposits which form along fractures and faults as open-space fillings or replacements. They are generally polymetallic. Many have been mined for copper, lead and zinc, although substantial gold and silver credits occur locally. These deposits generally fall in the category of low tonnage, high grade types of deposits. There are two broad categories: 1) vein deposits associated with porphyry base metal deposits, and 2) vein deposits not associated with porphyry base metal deposits. Zoned vein deposits which are associated with porphyry base metal deposits appear to form at lower temperatures during a later mineralization event. These veins are characterized by a strong sense of zoning from high temperature minerals in proximal (closer to the pluton) portions of the veins, to low temperature minerals in distal (far away) portions of the veins. Proximal portions of the veins are copper-rich and contain sulfide minerals with high metal: sulfur ratios. Distal portions of the same veins are lead-zinc-rich and contain sulfide minerals with lower metal: sulfur ratios. At Butte, Montana, alteration halos adjacent to the veins change dramatically along the length of the vein and with increasing distance from the central porphyry copper-molybdenum deposit (Figure 8 – 2). Proximal portions of the veins are characterized by advanced argillic alteration adjacent to the vein which is superceded outwards by sericitic alteration. Distal portions of the veins are characterized by propylitic alteration adjacent to the vein which gives rise to fresh unaltered rock further away from the vein. Zoned vein deposits which are not associated with porphyry base metal deposits are characterized by having moderate, more uniform temperatures over a larger area. Zoning in these types of vein deposits is usually a function changes in the fugacity of sulfur along the length of the vein.
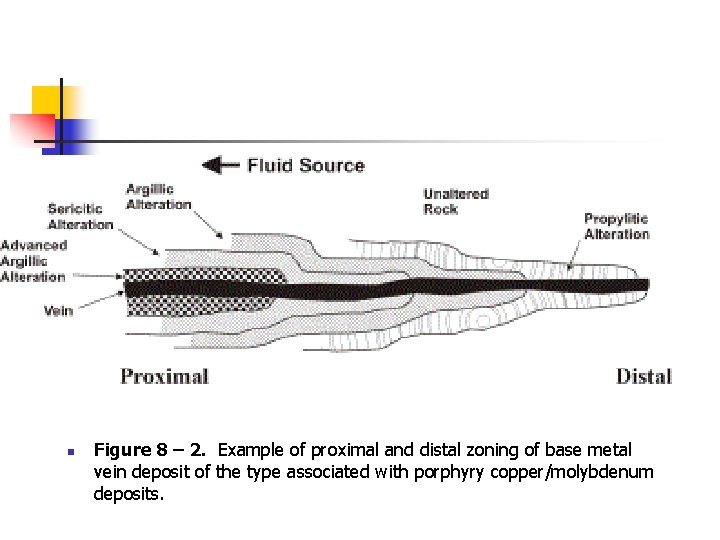
n Figure 8 – 2. Example of proximal and distal zoning of base metal vein deposit of the type associated with porphyry copper/molybdenum deposits.
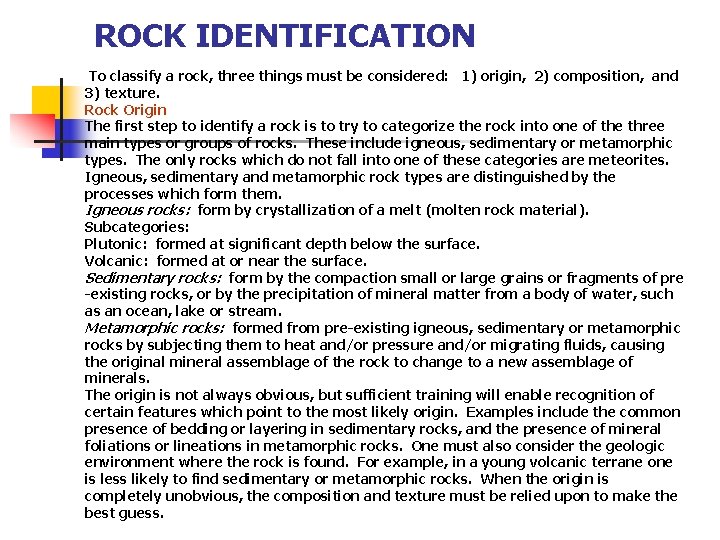
ROCK IDENTIFICATION To classify a rock, three things must be considered: 1) origin, 2) composition, and 3) texture. Rock Origin The first step to identify a rock is to try to categorize the rock into one of the three main types or groups of rocks. These include igneous, sedimentary or metamorphic types. The only rocks which do not fall into one of these categories are meteorites. Igneous, sedimentary and metamorphic rock types are distinguished by the processes which form them. Igneous rocks: form by crystallization of a melt (molten rock material). Subcategories: Plutonic: formed at significant depth below the surface. Volcanic: formed at or near the surface. Sedimentary rocks: form by the compaction small or large grains or fragments of pre -existing rocks, or by the precipitation of mineral matter from a body of water, such as an ocean, lake or stream. Metamorphic rocks: formed from pre-existing igneous, sedimentary or metamorphic rocks by subjecting them to heat and/or pressure and/or migrating fluids, causing the original mineral assemblage of the rock to change to a new assemblage of minerals. The origin is not always obvious, but sufficient training will enable recognition of certain features which point to the most likely origin. Examples include the common presence of bedding or layering in sedimentary rocks, and the presence of mineral foliations or lineations in metamorphic rocks. One must also consider the geologic environment where the rock is found. For example, in a young volcanic terrane one is less likely to find sedimentary or metamorphic rocks. When the origin is completely unobvious, the composition and texture must be relied upon to make the best guess.
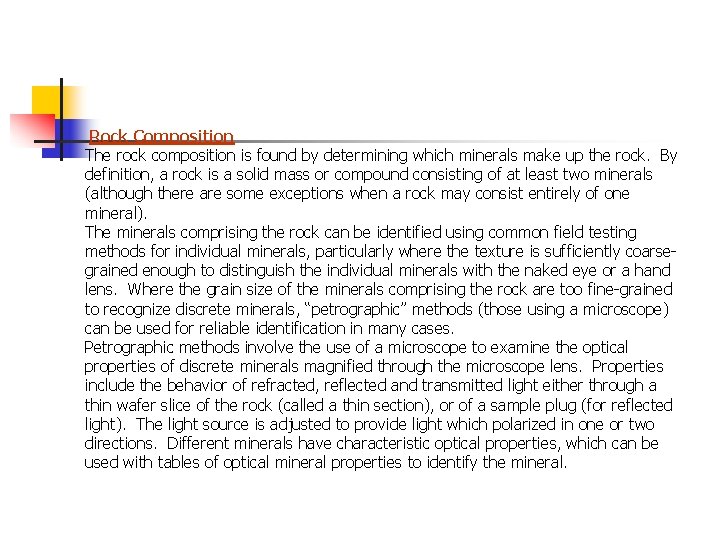
Rock Composition The rock composition is found by determining which minerals make up the rock. By definition, a rock is a solid mass or compound consisting of at least two minerals (although there are some exceptions when a rock may consist entirely of one mineral). The minerals comprising the rock can be identified using common field testing methods for individual minerals, particularly where the texture is sufficiently coarsegrained enough to distinguish the individual minerals with the naked eye or a hand lens. Where the grain size of the minerals comprising the rock are too fine-grained to recognize discrete minerals, “petrographic” methods (those using a microscope) can be used for reliable identification in many cases. Petrographic methods involve the use of a microscope to examine the optical properties of discrete minerals magnified through the microscope lens. Properties include the behavior of refracted, reflected and transmitted light either through a thin wafer slice of the rock (called a thin section), or of a sample plug (for reflected light). The light source is adjusted to provide light which polarized in one or two directions. Different minerals have characteristic optical properties, which can be used with tables of optical mineral properties to identify the mineral.
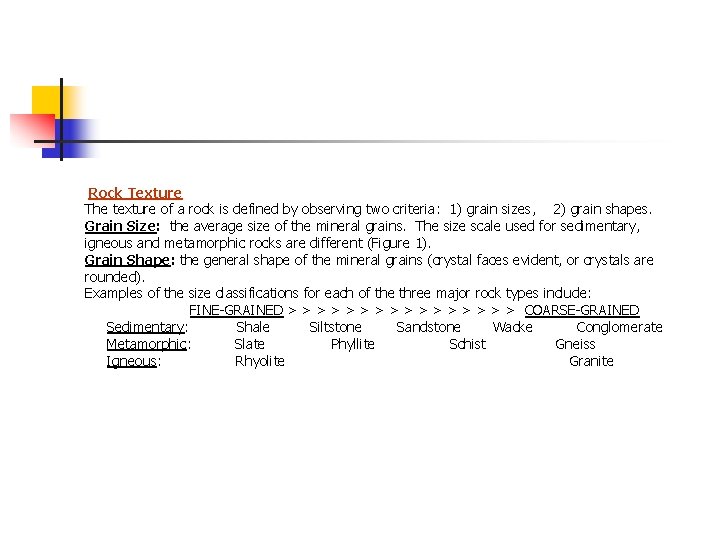
Rock Texture The texture of a rock is defined by observing two criteria: 1) grain sizes, 2) grain shapes. Grain Size: the average size of the mineral grains. The size scale used for sedimentary, igneous and metamorphic rocks are different (Figure 1). Grain Shape: the general shape of the mineral grains (crystal faces evident, or crystals are rounded). Examples of the size classifications for each of the three major rock types include: FINE-GRAINED > > > > COARSE-GRAINED Sedimentary: Shale Siltstone Sandstone Wacke Conglomerate Metamorphic: Slate Phyllite Schist Gneiss Igneous: Rhyolite Granite
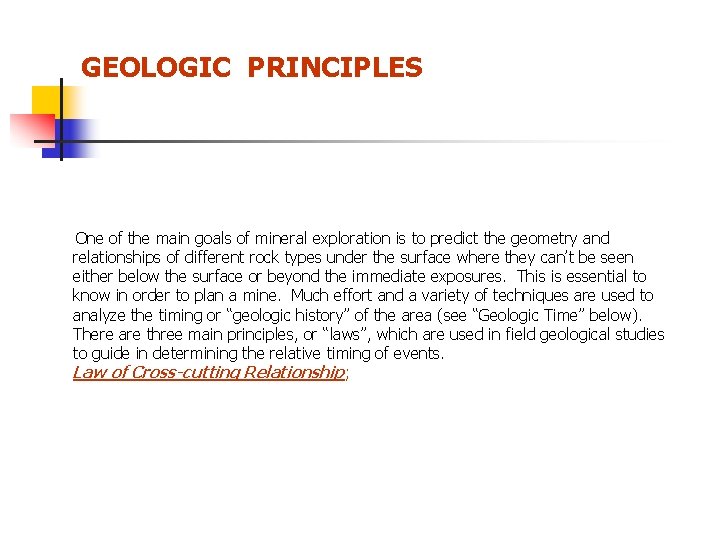
GEOLOGIC PRINCIPLES One of the main goals of mineral exploration is to predict the geometry and relationships of different rock types under the surface where they can’t be seen either below the surface or beyond the immediate exposures. This is essential to know in order to plan a mine. Much effort and a variety of techniques are used to analyze the timing or “geologic history” of the area (see “Geologic Time” below). There are three main principles, or “laws”, which are used in field geological studies to guide in determining the relative timing of events. Law of Cross-cutting Relationship;
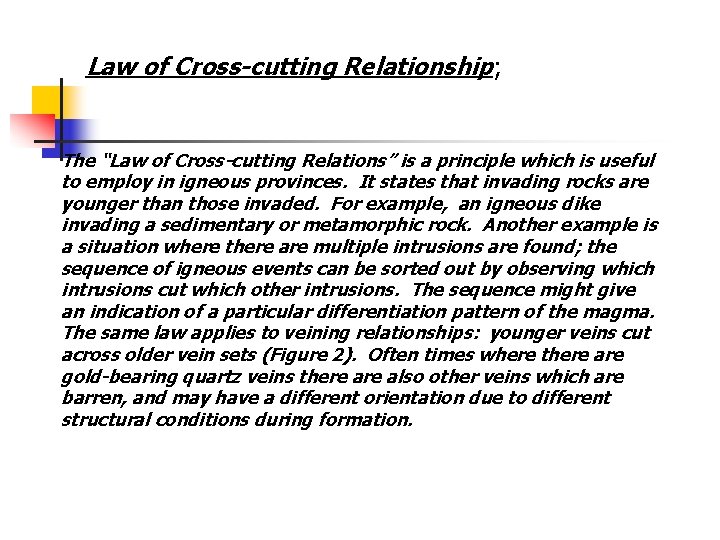
Law of Cross-cutting Relationship; The “Law of Cross-cutting Relations” is a principle which is useful to employ in igneous provinces. It states that invading rocks are younger than those invaded. For example, an igneous dike invading a sedimentary or metamorphic rock. Another example is a situation where there are multiple intrusions are found; the sequence of igneous events can be sorted out by observing which intrusions cut which other intrusions. The sequence might give an indication of a particular differentiation pattern of the magma. The same law applies to veining relationships: younger veins cut across older vein sets (Figure 2). Often times where there are gold-bearing quartz veins there also other veins which are barren, and may have a different orientation due to different structural conditions during formation.
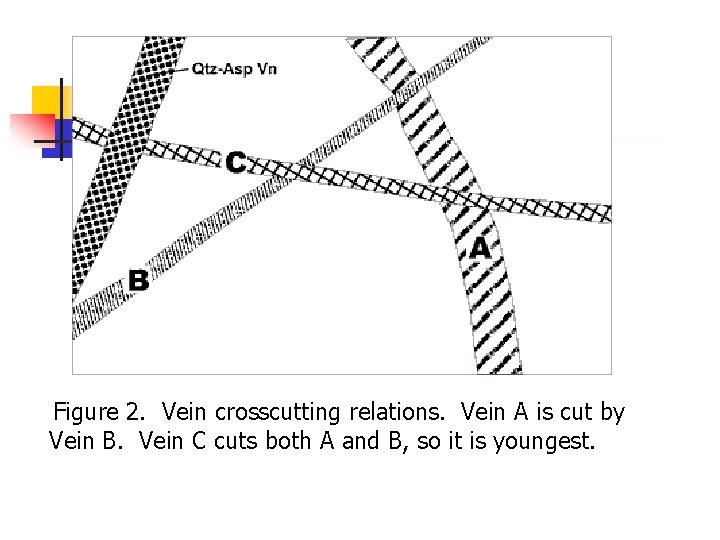
Figure 2. Vein crosscutting relations. Vein A is cut by Vein B. Vein C cuts both A and B, so it is youngest.
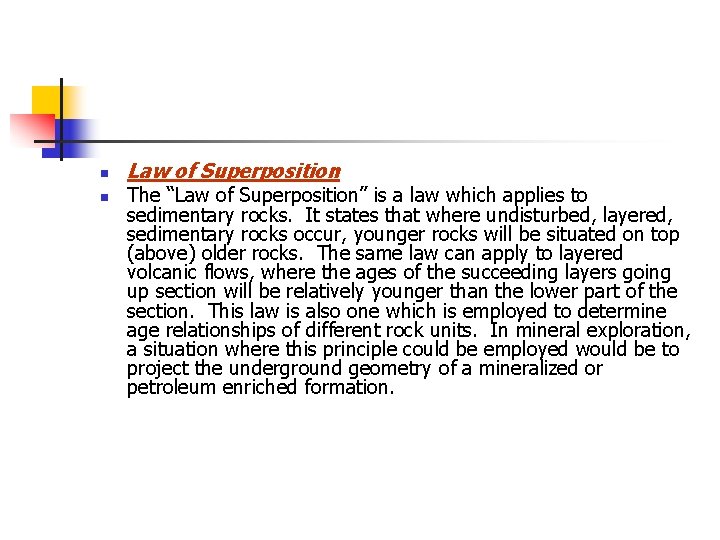
n n Law of Superposition The “Law of Superposition” is a law which applies to sedimentary rocks. It states that where undisturbed, layered, sedimentary rocks occur, younger rocks will be situated on top (above) older rocks. The same law can apply to layered volcanic flows, where the ages of the succeeding layers going up section will be relatively younger than the lower part of the section. This law is also one which is employed to determine age relationships of different rock units. In mineral exploration, a situation where this principle could be employed would be to project the underground geometry of a mineralized or petroleum enriched formation.
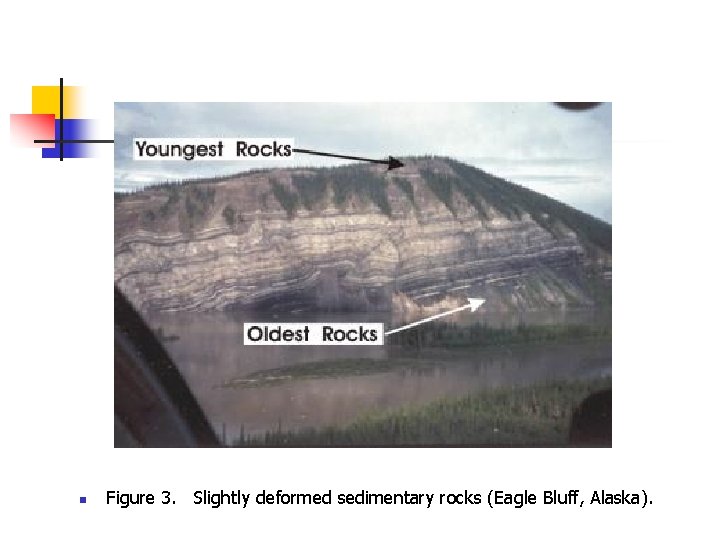
n Figure 3. Slightly deformed sedimentary rocks (Eagle Bluff, Alaska).
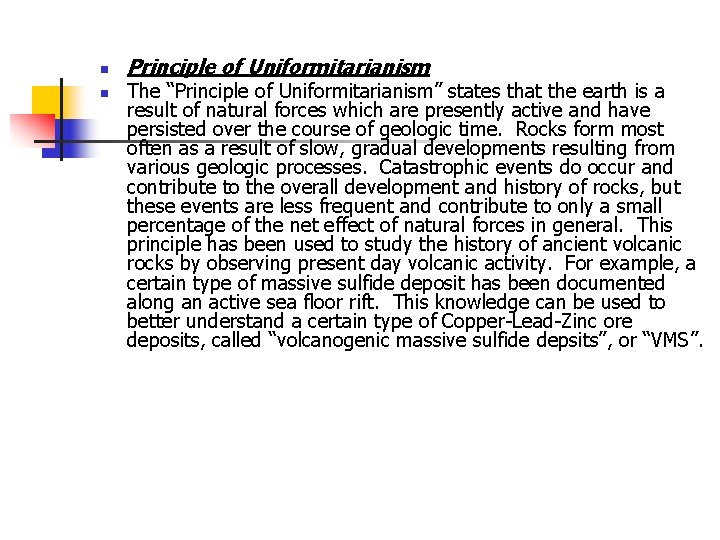
n n Principle of Uniformitarianism The “Principle of Uniformitarianism” states that the earth is a result of natural forces which are presently active and have persisted over the course of geologic time. Rocks form most often as a result of slow, gradual developments resulting from various geologic processes. Catastrophic events do occur and contribute to the overall development and history of rocks, but these events are less frequent and contribute to only a small percentage of the net effect of natural forces in general. This principle has been used to study the history of ancient volcanic rocks by observing present day volcanic activity. For example, a certain type of massive sulfide deposit has been documented along an active sea floor rift. This knowledge can be used to better understand a certain type of Copper-Lead-Zinc ore deposits, called “volcanogenic massive sulfide depsits”, or “VMS”.
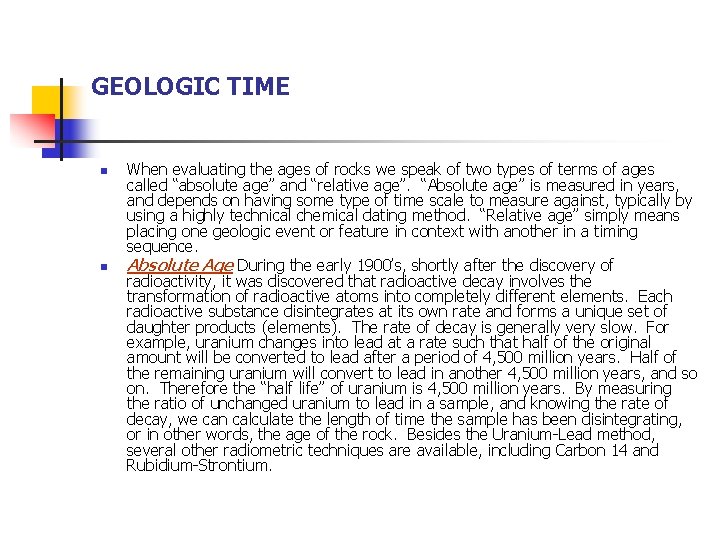
GEOLOGIC TIME n n When evaluating the ages of rocks we speak of two types of terms of ages called “absolute age” and “relative age”. “Absolute age” is measured in years, and depends on having some type of time scale to measure against, typically by using a highly technical chemical dating method. “Relative age” simply means placing one geologic event or feature in context with another in a timing sequence. Absolute Age During the early 1900’s, shortly after the discovery of radioactivity, it was discovered that radioactive decay involves the transformation of radioactive atoms into completely different elements. Each radioactive substance disintegrates at its own rate and forms a unique set of daughter products (elements). The rate of decay is generally very slow. For example, uranium changes into lead at a rate such that half of the original amount will be converted to lead after a period of 4, 500 million years. Half of the remaining uranium will convert to lead in another 4, 500 million years, and so on. Therefore the “half life” of uranium is 4, 500 million years. By measuring the ratio of unchanged uranium to lead in a sample, and knowing the rate of decay, we can calculate the length of time the sample has been disintegrating, or in other words, the age of the rock. Besides the Uranium-Lead method, several other radiometric techniques are available, including Carbon 14 and Rubidium-Strontium.
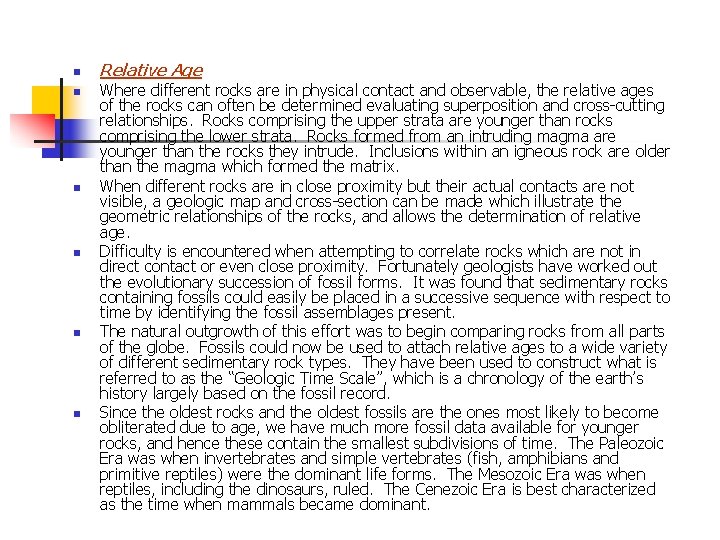
n n n Relative Age Where different rocks are in physical contact and observable, the relative ages of the rocks can often be determined evaluating superposition and cross-cutting relationships. Rocks comprising the upper strata are younger than rocks comprising the lower strata. Rocks formed from an intruding magma are younger than the rocks they intrude. Inclusions within an igneous rock are older than the magma which formed the matrix. When different rocks are in close proximity but their actual contacts are not visible, a geologic map and cross-section can be made which illustrate the geometric relationships of the rocks, and allows the determination of relative age. Difficulty is encountered when attempting to correlate rocks which are not in direct contact or even close proximity. Fortunately geologists have worked out the evolutionary succession of fossil forms. It was found that sedimentary rocks containing fossils could easily be placed in a successive sequence with respect to time by identifying the fossil assemblages present. The natural outgrowth of this effort was to begin comparing rocks from all parts of the globe. Fossils could now be used to attach relative ages to a wide variety of different sedimentary rock types. They have been used to construct what is referred to as the “Geologic Time Scale”, which is a chronology of the earth’s history largely based on the fossil record. Since the oldest rocks and the oldest fossils are the ones most likely to become obliterated due to age, we have much more fossil data available for younger rocks, and hence these contain the smallest subdivisions of time. The Paleozoic Era was when invertebrates and simple vertebrates (fish, amphibians and primitive reptiles) were the dominant life forms. The Mesozoic Era was when reptiles, including the dinosaurs, ruled. The Cenezoic Era is best characterized as the time when mammals became dominant.
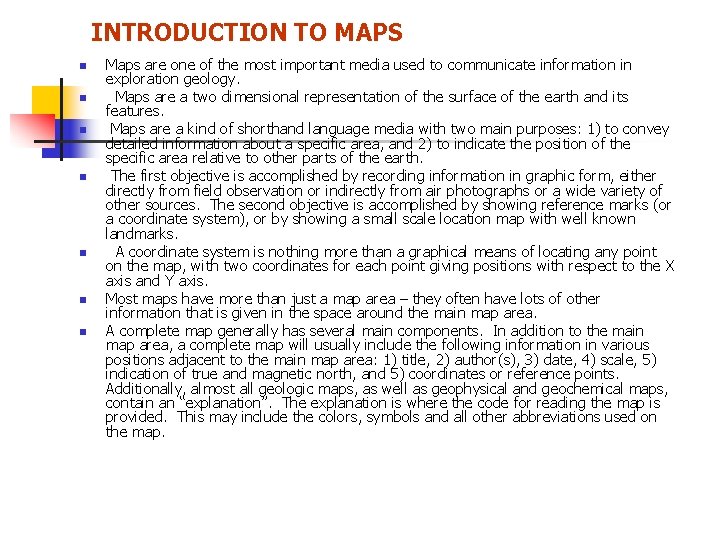
INTRODUCTION TO MAPS n n n n Maps are one of the most important media used to communicate information in exploration geology. Maps are a two dimensional representation of the surface of the earth and its features. Maps are a kind of shorthand language media with two main purposes: 1) to convey detailed information about a specific area, and 2) to indicate the position of the specific area relative to other parts of the earth. The first objective is accomplished by recording information in graphic form, either directly from field observation or indirectly from air photographs or a wide variety of other sources. The second objective is accomplished by showing reference marks (or a coordinate system), or by showing a small scale location map with well known landmarks. A coordinate system is nothing more than a graphical means of locating any point on the map, with two coordinates for each point giving positions with respect to the X axis and Y axis. Most maps have more than just a map area – they often have lots of other information that is given in the space around the main map area. A complete map generally has several main components. In addition to the main map area, a complete map will usually include the following information in various positions adjacent to the main map area: 1) title, 2) author(s), 3) date, 4) scale, 5) indication of true and magnetic north, and 5) coordinates or reference points. Additionally, almost all geologic maps, as well as geophysical and geochemical maps, contain an “explanation”. The explanation is where the code for reading the map is provided. This may include the colors, symbols and all other abbreviations used on the map.
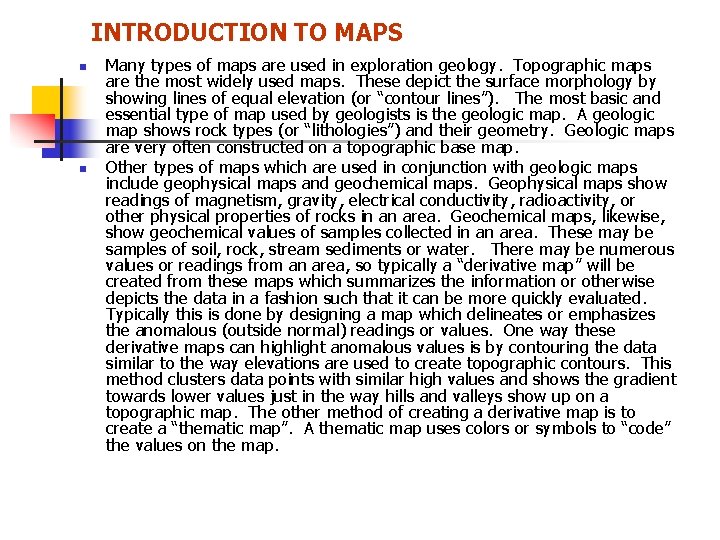
INTRODUCTION TO MAPS n n Many types of maps are used in exploration geology. Topographic maps are the most widely used maps. These depict the surface morphology by showing lines of equal elevation (or “contour lines”). The most basic and essential type of map used by geologists is the geologic map. A geologic map shows rock types (or “lithologies”) and their geometry. Geologic maps are very often constructed on a topographic base map. Other types of maps which are used in conjunction with geologic maps include geophysical maps and geochemical maps. Geophysical maps show readings of magnetism, gravity, electrical conductivity, radioactivity, or other physical properties of rocks in an area. Geochemical maps, likewise, show geochemical values of samples collected in an area. These may be samples of soil, rock, stream sediments or water. There may be numerous values or readings from an area, so typically a “derivative map” will be created from these maps which summarizes the information or otherwise depicts the data in a fashion such that it can be more quickly evaluated. Typically this is done by designing a map which delineates or emphasizes the anomalous (outside normal) readings or values. One way these derivative maps can highlight anomalous values is by contouring the data similar to the way elevations are used to create topographic contours. This method clusters data points with similar high values and shows the gradient towards lower values just in the way hills and valleys show up on a topographic map. The other method of creating a derivative map is to create a “thematic map”. A thematic map uses colors or symbols to “code” the values on the map.
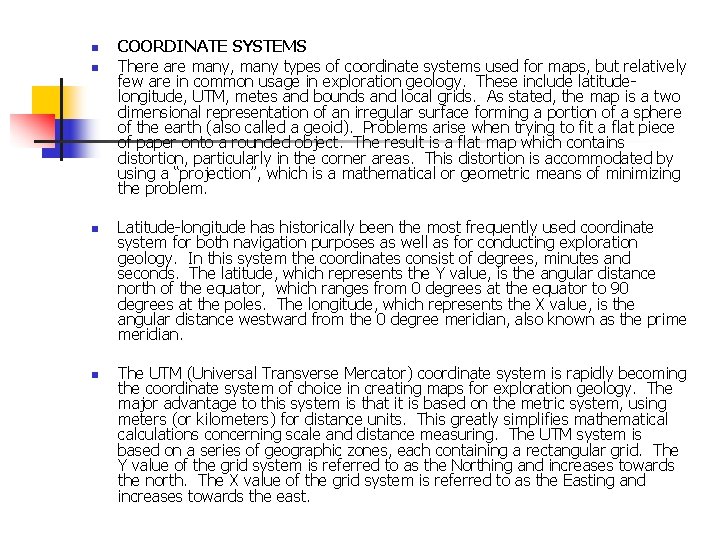
n n COORDINATE SYSTEMS There are many, many types of coordinate systems used for maps, but relatively few are in common usage in exploration geology. These include latitudelongitude, UTM, metes and bounds and local grids. As stated, the map is a two dimensional representation of an irregular surface forming a portion of a sphere of the earth (also called a geoid). Problems arise when trying to fit a flat piece of paper onto a rounded object. The result is a flat map which contains distortion, particularly in the corner areas. This distortion is accommodated by using a “projection”, which is a mathematical or geometric means of minimizing the problem. Latitude-longitude has historically been the most frequently used coordinate system for both navigation purposes as well as for conducting exploration geology. In this system the coordinates consist of degrees, minutes and seconds. The latitude, which represents the Y value, is the angular distance north of the equator, which ranges from 0 degrees at the equator to 90 degrees at the poles. The longitude, which represents the X value, is the angular distance westward from the 0 degree meridian, also known as the prime meridian. The UTM (Universal Transverse Mercator) coordinate system is rapidly becoming the coordinate system of choice in creating maps for exploration geology. The major advantage to this system is that it is based on the metric system, using meters (or kilometers) for distance units. This greatly simplifies mathematical calculations concerning scale and distance measuring. The UTM system is based on a series of geographic zones, each containing a rectangular grid. The Y value of the grid system is referred to as the Northing and increases towards the north. The X value of the grid system is referred to as the Easting and increases towards the east.
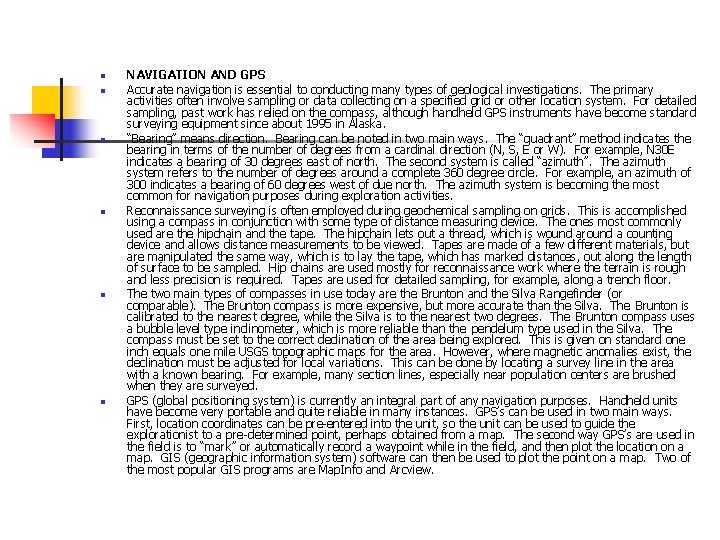
n n n NAVIGATION AND GPS Accurate navigation is essential to conducting many types of geological investigations. The primary activities often involve sampling or data collecting on a specified grid or other location system. For detailed sampling, past work has relied on the compass, although handheld GPS instruments have become standard surveying equipment since about 1995 in Alaska. “Bearing” means direction. Bearing can be noted in two main ways. The “quadrant” method indicates the bearing in terms of the number of degrees from a cardinal direction (N, S, E or W). For example, N 30 E indicates a bearing of 30 degrees east of north. The second system is called “azimuth”. The azimuth system refers to the number of degrees around a complete 360 degree circle. For example, an azimuth of 300 indicates a bearing of 60 degrees west of due north. The azimuth system is becoming the most common for navigation purposes during exploration activities. Reconnaissance surveying is often employed during geochemical sampling on grids. This is accomplished using a compass in conjunction with some type of distance measuring device. The ones most commonly used are the hipchain and the tape. The hipchain lets out a thread, which is wound around a counting device and allows distance measurements to be viewed. Tapes are made of a few different materials, but are manipulated the same way, which is to lay the tape, which has marked distances, out along the length of surface to be sampled. Hip chains are used mostly for reconnaissance work where the terrain is rough and less precision is required. Tapes are used for detailed sampling, for example, along a trench floor. The two main types of compasses in use today are the Brunton and the Silva Rangefinder (or comparable). The Brunton compass is more expensive, but more accurate than the Silva. The Brunton is calibrated to the nearest degree, while the Silva is to the nearest two degrees. The Brunton compass uses a bubble level type inclinometer, which is more reliable than the pendelum type used in the Silva. The compass must be set to the correct declination of the area being explored. This is given on standard one inch equals one mile USGS topographic maps for the area. However, where magnetic anomalies exist, the declination must be adjusted for local variations. This can be done by locating a survey line in the area with a known bearing. For example, many section lines, especially near population centers are brushed when they are surveyed. GPS (global positioning system) is currently an integral part of any navigation purposes. Handheld units have become very portable and quite reliable in many instances. GPS’s can be used in two main ways. First, location coordinates can be pre-entered into the unit, so the unit can be used to guide the explorationist to a pre-determined point, perhaps obtained from a map. The second way GPS’s are used in the field is to “mark” or automatically record a waypoint while in the field, and then plot the location on a map. GIS (geographic information system) software can then be used to plot the point on a map. Two of the most popular GIS programs are Map. Info and Arcview.
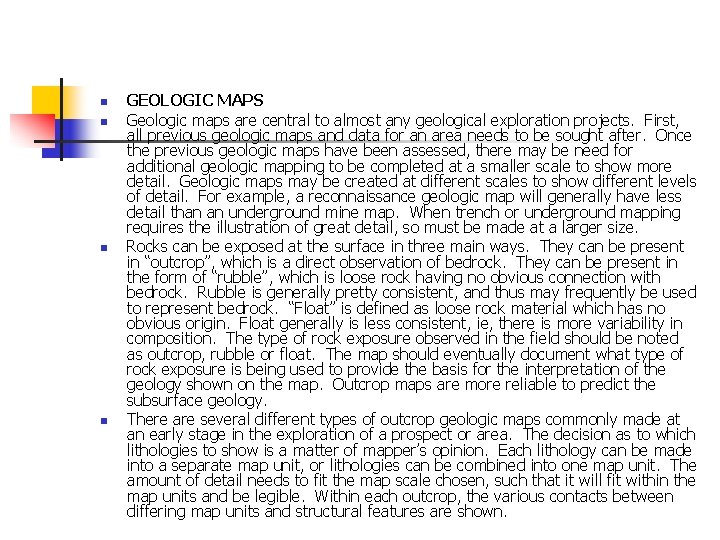
n n GEOLOGIC MAPS Geologic maps are central to almost any geological exploration projects. First, all previous geologic maps and data for an area needs to be sought after. Once the previous geologic maps have been assessed, there may be need for additional geologic mapping to be completed at a smaller scale to show more detail. Geologic maps may be created at different scales to show different levels of detail. For example, a reconnaissance geologic map will generally have less detail than an underground mine map. When trench or underground mapping requires the illustration of great detail, so must be made at a larger size. Rocks can be exposed at the surface in three main ways. They can be present in “outcrop”, which is a direct observation of bedrock. They can be present in the form of “rubble”, which is loose rock having no obvious connection with bedrock. Rubble is generally pretty consistent, and thus may frequently be used to represent bedrock. “Float” is defined as loose rock material which has no obvious origin. Float generally is less consistent, ie, there is more variability in composition. The type of rock exposure observed in the field should be noted as outcrop, rubble or float. The map should eventually document what type of rock exposure is being used to provide the basis for the interpretation of the geology shown on the map. Outcrop maps are more reliable to predict the subsurface geology. There are several different types of outcrop geologic maps commonly made at an early stage in the exploration of a prospect or area. The decision as to which lithologies to show is a matter of mapper’s opinion. Each lithology can be made into a separate map unit, or lithologies can be combined into one map unit. The amount of detail needs to fit the map scale chosen, such that it will fit within the map units and be legible. Within each outcrop, the various contacts between differing map units and structural features are shown.
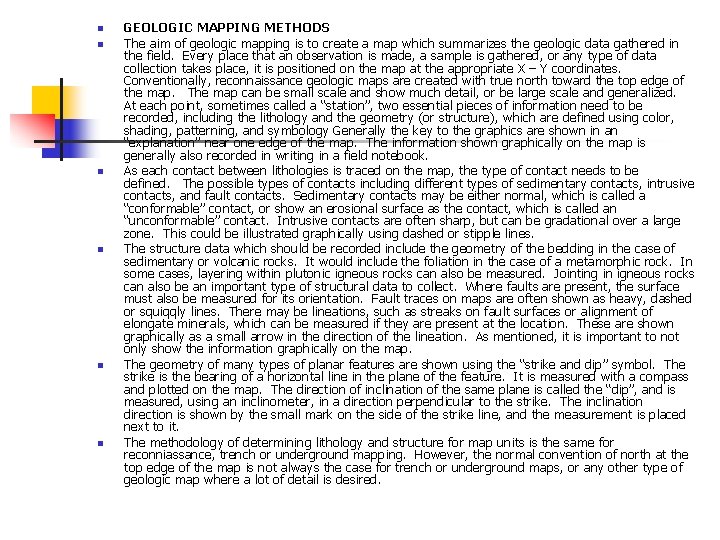
n n n GEOLOGIC MAPPING METHODS The aim of geologic mapping is to create a map which summarizes the geologic data gathered in the field. Every place that an observation is made, a sample is gathered, or any type of data collection takes place, it is positioned on the map at the appropriate X – Y coordinates. Conventionally, reconnaissance geologic maps are created with true north toward the top edge of the map. The map can be small scale and show much detail, or be large scale and generalized. At each point, sometimes called a “station”, two essential pieces of information need to be recorded, including the lithology and the geometry (or structure), which are defined using color, shading, patterning, and symbology Generally the key to the graphics are shown in an “explanation” near one edge of the map. The information shown graphically on the map is generally also recorded in writing in a field notebook. As each contact between lithologies is traced on the map, the type of contact needs to be defined. The possible types of contacts including different types of sedimentary contacts, intrusive contacts, and fault contacts. Sedimentary contacts may be either normal, which is called a “conformable” contact, or show an erosional surface as the contact, which is called an “unconformable” contact. Intrusive contacts are often sharp, but can be gradational over a large zone. This could be illustrated graphically using dashed or stipple lines. The structure data which should be recorded include the geometry of the bedding in the case of sedimentary or volcanic rocks. It would include the foliation in the case of a metamorphic rock. In some cases, layering within plutonic igneous rocks can also be measured. Jointing in igneous rocks can also be an important type of structural data to collect. Where faults are present, the surface must also be measured for its orientation. Fault traces on maps are often shown as heavy, dashed or squiqqly lines. There may be lineations, such as streaks on fault surfaces or alignment of elongate minerals, which can be measured if they are present at the location. These are shown graphically as a small arrow in the direction of the lineation. As mentioned, it is important to not only show the information graphically on the map. The geometry of many types of planar features are shown using the “strike and dip” symbol. The strike is the bearing of a horizontal line in the plane of the feature. It is measured with a compass and plotted on the map. The direction of inclination of the same plane is called the “dip”, and is measured, using an inclinometer, in a direction perpendicular to the strike. The inclination direction is shown by the small mark on the side of the strike line, and the measurement is placed next to it. The methodology of determining lithology and structure for map units is the same for reconniassance, trench or underground mapping. However, the normal convention of north at the top edge of the map is not always the case for trench or underground maps, or any other type of geologic map where a lot of detail is desired.
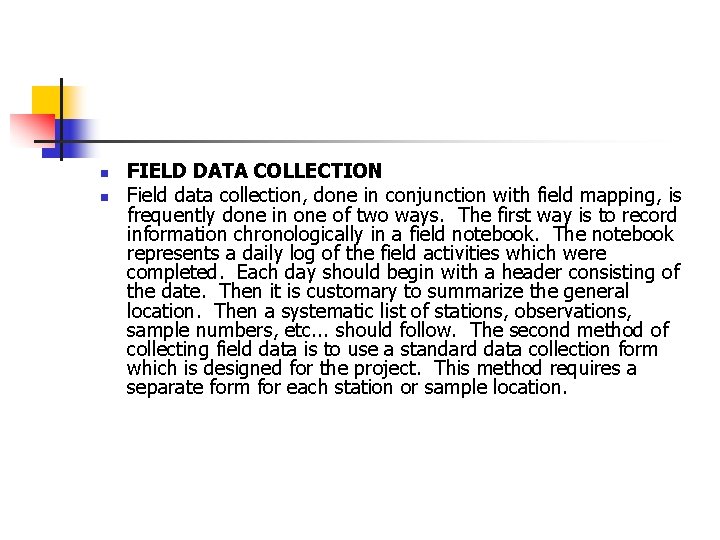
n n FIELD DATA COLLECTION Field data collection, done in conjunction with field mapping, is frequently done in one of two ways. The first way is to record information chronologically in a field notebook. The notebook represents a daily log of the field activities which were completed. Each day should begin with a header consisting of the date. Then it is customary to summarize the general location. Then a systematic list of stations, observations, sample numbers, etc. . . should follow. The second method of collecting field data is to use a standard data collection form which is designed for the project. This method requires a separate form for each station or sample location.
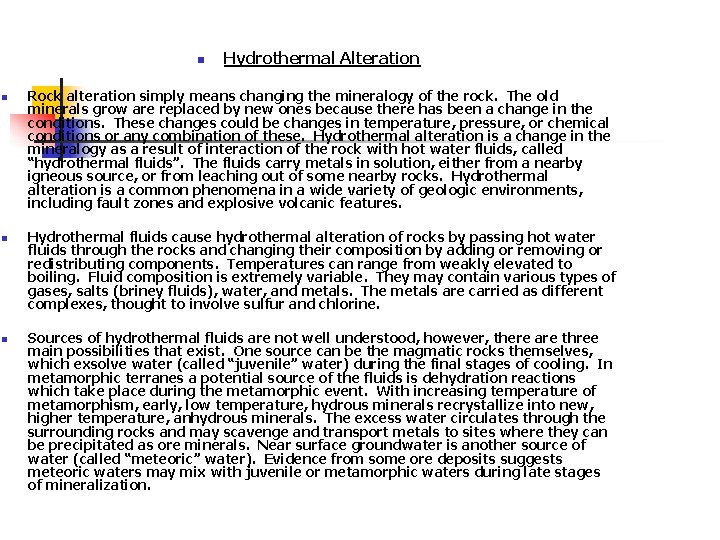
n n Hydrothermal Alteration Rock alteration simply means changing the mineralogy of the rock. The old minerals grow are replaced by new ones because there has been a change in the conditions. These changes could be changes in temperature, pressure, or chemical conditions or any combination of these. Hydrothermal alteration is a change in the mineralogy as a result of interaction of the rock with hot water fluids, called “hydrothermal fluids”. The fluids carry metals in solution, either from a nearby igneous source, or from leaching out of some nearby rocks. Hydrothermal alteration is a common phenomena in a wide variety of geologic environments, including fault zones and explosive volcanic features. Hydrothermal fluids cause hydrothermal alteration of rocks by passing hot water fluids through the rocks and changing their composition by adding or removing or redistributing components. Temperatures can range from weakly elevated to boiling. Fluid composition is extremely variable. They may contain various types of gases, salts (briney fluids), water, and metals. The metals are carried as different complexes, thought to involve sulfur and chlorine. Sources of hydrothermal fluids are not well understood, however, there are three main possibilities that exist. One source can be the magmatic rocks themselves, which exsolve water (called “juvenile” water) during the final stages of cooling. In metamorphic terranes a potential source of the fluids is dehydration reactions which take place during the metamorphic event. With increasing temperature of metamorphism, early, low temperature, hydrous minerals recrystallize into new, higher temperature, anhydrous minerals. The excess water circulates through the surrounding rocks and may scavenge and transport metals to sites where they can be precipitated as ore minerals. Near surface groundwater is another source of water (called “meteoric” water). Evidence from some ore deposits suggests meteoric waters may mix with juvenile or metamorphic waters during late stages of mineralization.
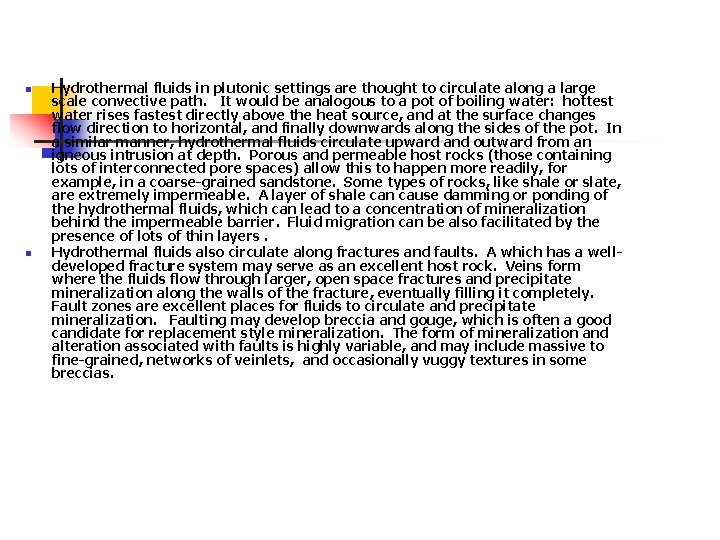
n n Hydrothermal fluids in plutonic settings are thought to circulate along a large scale convective path. It would be analogous to a pot of boiling water: hottest water rises fastest directly above the heat source, and at the surface changes flow direction to horizontal, and finally downwards along the sides of the pot. In a similar manner, hydrothermal fluids circulate upward and outward from an igneous intrusion at depth. Porous and permeable host rocks (those containing lots of interconnected pore spaces) allow this to happen more readily, for example, in a coarse-grained sandstone. Some types of rocks, like shale or slate, are extremely impermeable. A layer of shale can cause damming or ponding of the hydrothermal fluids, which can lead to a concentration of mineralization behind the impermeable barrier. Fluid migration can be also facilitated by the presence of lots of thin layers. Hydrothermal fluids also circulate along fractures and faults. A which has a welldeveloped fracture system may serve as an excellent host rock. Veins form where the fluids flow through larger, open space fractures and precipitate mineralization along the walls of the fracture, eventually filling it completely. Fault zones are excellent places for fluids to circulate and precipitate mineralization. Faulting may develop breccia and gouge, which is often a good candidate for replacement style mineralization. The form of mineralization and alteration associated with faults is highly variable, and may include massive to fine-grained, networks of veinlets, and occasionally vuggy textures in some breccias.
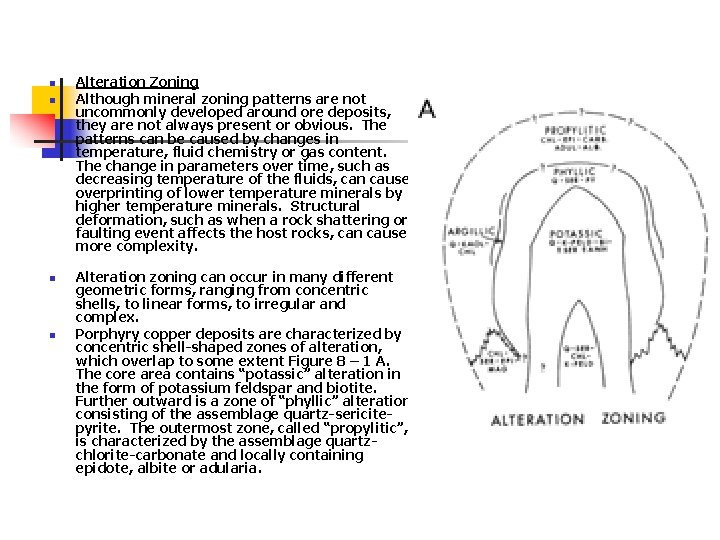
n n Alteration Zoning Although mineral zoning patterns are not uncommonly developed around ore deposits, they are not always present or obvious. The patterns can be caused by changes in temperature, fluid chemistry or gas content. The change in parameters over time, such as decreasing temperature of the fluids, can cause overprinting of lower temperature minerals by higher temperature minerals. Structural deformation, such as when a rock shattering or faulting event affects the host rocks, can cause more complexity. Alteration zoning can occur in many different geometric forms, ranging from concentric shells, to linear forms, to irregular and complex. Porphyry copper deposits are characterized by concentric shell-shaped zones of alteration, which overlap to some extent Figure 8 – 1 A. The core area contains “potassic” alteration in the form of potassium feldspar and biotite. Further outward is a zone of “phyllic” alteration consisting of the assemblage quartz-sericitepyrite. The outermost zone, called “propylitic”, is characterized by the assemblage quartzchlorite-carbonate and locally containing epidote, albite or adularia.
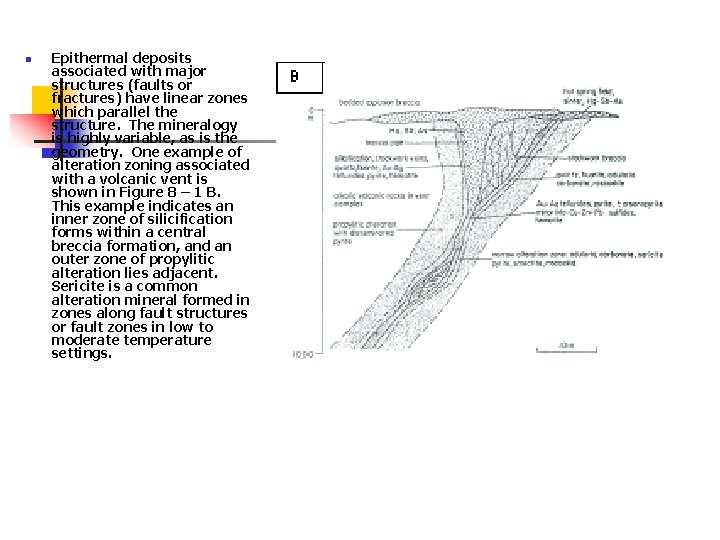
n Epithermal deposits associated with major structures (faults or fractures) have linear zones which parallel the structure. The mineralogy is highly variable, as is the geometry. One example of alteration zoning associated with a volcanic vent is shown in Figure 8 – 1 B. This example indicates an inner zone of silicification forms within a central breccia formation, and an outer zone of propylitic alteration lies adjacent. Sericite is a common alteration mineral formed in zones along fault structures or fault zones in low to moderate temperature settings.
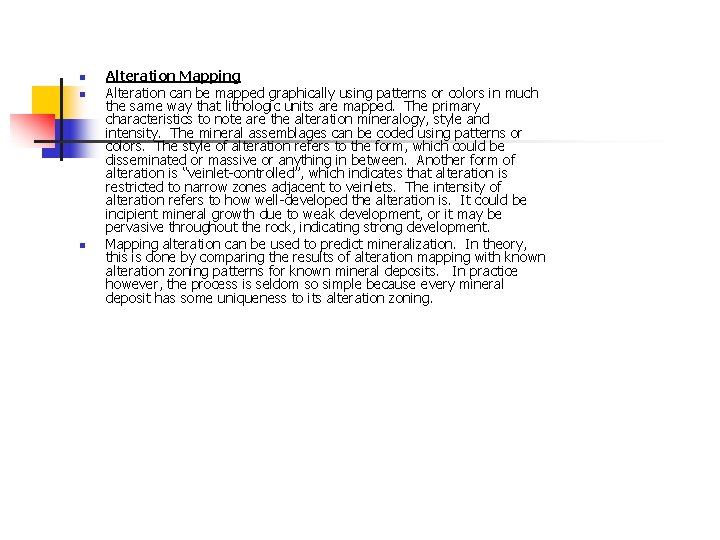
n n n Alteration Mapping Alteration can be mapped graphically using patterns or colors in much the same way that lithologic units are mapped. The primary characteristics to note are the alteration mineralogy, style and intensity. The mineral assemblages can be coded using patterns or colors. The style of alteration refers to the form, which could be disseminated or massive or anything in between. Another form of alteration is “veinlet-controlled”, which indicates that alteration is restricted to narrow zones adjacent to veinlets. The intensity of alteration refers to how well-developed the alteration is. It could be incipient mineral growth due to weak development, or it may be pervasive throughout the rock, indicating strong development. Mapping alteration can be used to predict mineralization. In theory, this is done by comparing the results of alteration mapping with known alteration zoning patterns for known mineral deposits. In practice however, the process is seldom so simple because every mineral deposit has some uniqueness to its alteration zoning.
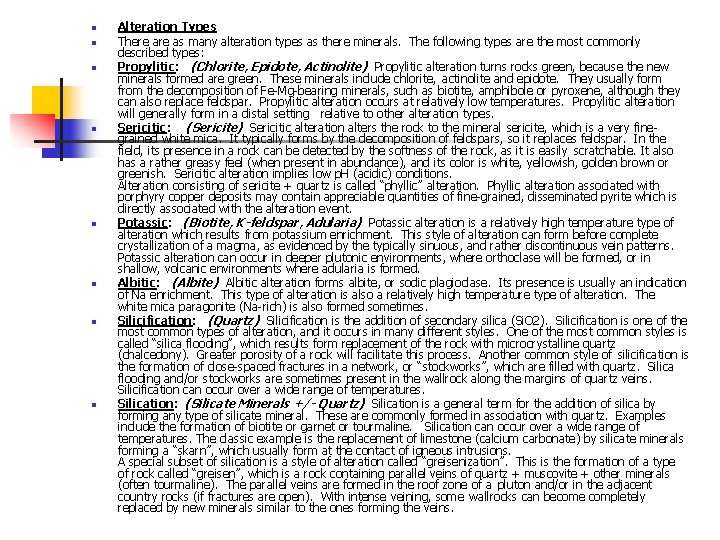
n n n n Alteration Types There as many alteration types as there minerals. The following types are the most commonly described types: Propylitic: (Chlorite, Epidote, Actinolite) Propylitic alteration turns rocks green, because the new minerals formed are green. These minerals include chlorite, actinolite and epidote. They usually form from the decomposition of Fe-Mg-bearing minerals, such as biotite, amphibole or pyroxene, although they can also replace feldspar. Propylitic alteration occurs at relatively low temperatures. Propylitic alteration will generally form in a distal setting relative to other alteration types. Sericitic: (Sericite) Sericitic alteration alters the rock to the mineral sericite, which is a very finegrained white mica. It typically forms by the decomposition of feldspars, so it replaces feldspar. In the field, its presence in a rock can be detected by the softness of the rock, as it is easily scratchable. It also has a rather greasy feel (when present in abundance), and its color is white, yellowish, golden brown or greenish. Sericitic alteration implies low p. H (acidic) conditions. Alteration consisting of sericite + quartz is called “phyllic” alteration. Phyllic alteration associated with porphyry copper deposits may contain appreciable quantities of fine-grained, disseminated pyrite which is directly associated with the alteration event. Potassic: (Biotite, K-feldspar, Adularia) Potassic alteration is a relatively high temperature type of alteration which results from potassium enrichment. This style of alteration can form before complete crystallization of a magma, as evidenced by the typically sinuous, and rather discontinuous vein patterns. Potassic alteration can occur in deeper plutonic environments, where orthoclase will be formed, or in shallow, volcanic environments where adularia is formed. Albitic: (Albite) Albitic alteration forms albite, or sodic plagioclase. Its presence is usually an indication of Na enrichment. This type of alteration is also a relatively high temperature type of alteration. The white mica paragonite (Na-rich) is also formed sometimes. Silicification: (Quartz) Silicification is the addition of secondary silica (Si. O 2). Silicification is one of the most common types of alteration, and it occurs in many different styles. One of the most common styles is called “silica flooding”, which results form replacement of the rock with microcrystalline quartz (chalcedony). Greater porosity of a rock will facilitate this process. Another common style of silicification is the formation of close-spaced fractures in a network, or “stockworks”, which are filled with quartz. Silica flooding and/or stockworks are sometimes present in the wallrock along the margins of quartz veins. Silicification can occur over a wide range of temperatures. Silication: (Silicate Minerals +/- Quartz) Silication is a general term for the addition of silica by forming any type of silicate mineral. These are commonly formed in association with quartz. Examples include the formation of biotite or garnet or tourmaline. Silication can occur over a wide range of temperatures. The classic example is the replacement of limestone (calcium carbonate) by silicate minerals forming a “skarn”, which usually form at the contact of igneous intrusions. A special subset of silication is a style of alteration called “greisenization”. This is the formation of a type of rock called “greisen”, which is a rock containing parallel veins of quartz + muscovite + other minerals (often tourmaline). The parallel veins are formed in the roof zone of a pluton and/or in the adjacent country rocks (if fractures are open). With intense veining, some wallrocks can become completely replaced by new minerals similar to the ones forming the veins.
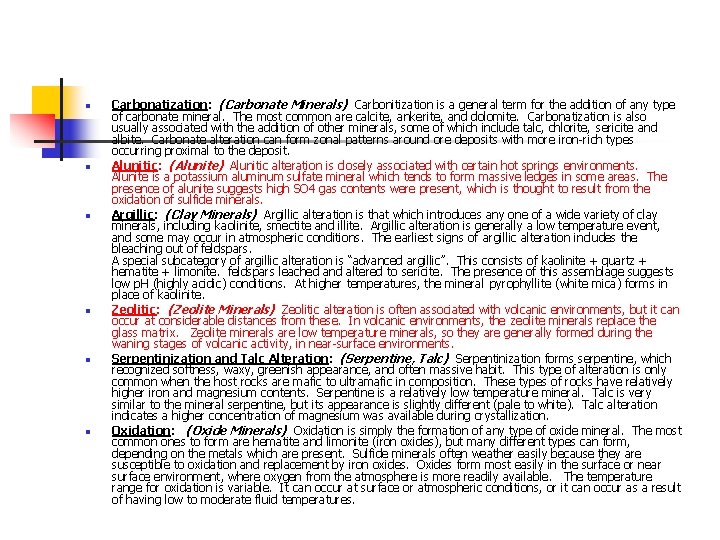
n n n Carbonatization: (Carbonate Minerals) Carbonitization is a general term for the addition of any type of carbonate mineral. The most common are calcite, ankerite, and dolomite. Carbonatization is also usually associated with the addition of other minerals, some of which include talc, chlorite, sericite and albite. Carbonate alteration can form zonal patterns around ore deposits with more iron-rich types occurring proximal to the deposit. Alunitic: (Alunite) Alunitic alteration is closely associated with certain hot springs environments. Alunite is a potassium aluminum sulfate mineral which tends to form massive ledges in some areas. The presence of alunite suggests high SO 4 gas contents were present, which is thought to result from the oxidation of sulfide minerals. Argillic: (Clay Minerals) Argillic alteration is that which introduces any one of a wide variety of clay minerals, including kaolinite, smectite and illite. Argillic alteration is generally a low temperature event, and some may occur in atmospheric conditions. The earliest signs of argillic alteration includes the bleaching out of feldspars. A special subcategory of argillic alteration is “advanced argillic”. This consists of kaolinite + quartz + hematite + limonite. feldspars leached and altered to sericite. The presence of this assemblage suggests low p. H (highly acidic) conditions. At higher temperatures, the mineral pyrophyllite (white mica) forms in place of kaolinite. Zeolitic: (Zeolite Minerals) Zeolitic alteration is often associated with volcanic environments, but it can occur at considerable distances from these. In volcanic environments, the zeolite minerals replace the glass matrix. Zeolite minerals are low temperature minerals, so they are generally formed during the waning stages of volcanic activity, in near-surface environments. Serpentinization and Talc Alteration: (Serpentine, Talc) Serpentinization forms serpentine, which recognized softness, waxy, greenish appearance, and often massive habit. This type of alteration is only common when the host rocks are mafic to ultramafic in composition. These types of rocks have relatively higher iron and magnesium contents. Serpentine is a relatively low temperature mineral. Talc is very similar to the mineral serpentine, but its appearance is slightly different (pale to white). Talc alteration indicates a higher concentration of magnesium was available during crystallization. Oxidation: (Oxide Minerals) Oxidation is simply the formation of any type of oxide mineral. The most common ones to form are hematite and limonite (iron oxides), but many different types can form, depending on the metals which are present. Sulfide minerals often weather easily because they are susceptible to oxidation and replacement by iron oxides. Oxides form most easily in the surface or near surface environment, where oxygen from the atmosphere is more readily available. The temperature range for oxidation is variable. It can occur at surface or atmospheric conditions, or it can occur as a result of having low to moderate fluid temperatures.
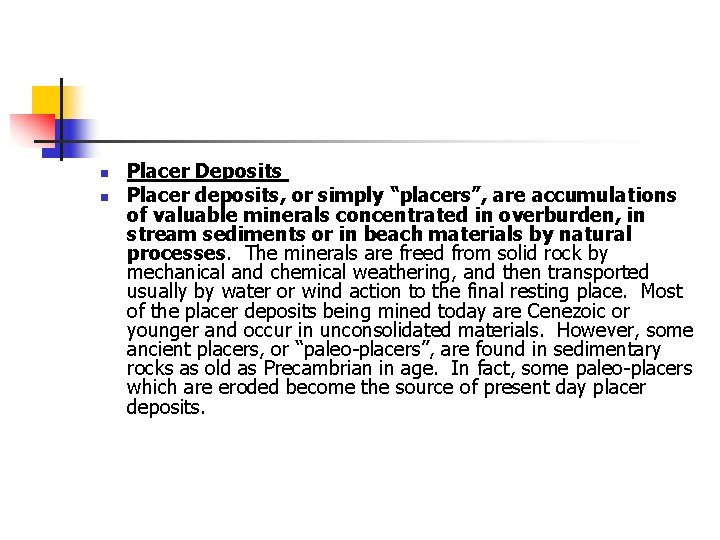
n n Placer Deposits Placer deposits, or simply “placers”, are accumulations of valuable minerals concentrated in overburden, in stream sediments or in beach materials by natural processes. The minerals are freed from solid rock by mechanical and chemical weathering, and then transported usually by water or wind action to the final resting place. Most of the placer deposits being mined today are Cenezoic or younger and occur in unconsolidated materials. However, some ancient placers, or “paleo-placers”, are found in sedimentary rocks as old as Precambrian in age. In fact, some paleo-placers which are eroded become the source of present day placer deposits.
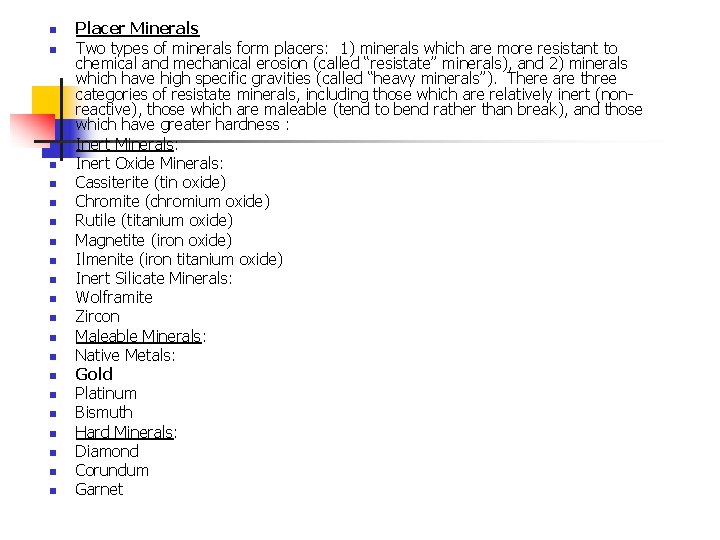
n n n n n n Placer Minerals Two types of minerals form placers: 1) minerals which are more resistant to chemical and mechanical erosion (called “resistate” minerals), and 2) minerals which have high specific gravities (called “heavy minerals”). There are three categories of resistate minerals, including those which are relatively inert (nonreactive), those which are maleable (tend to bend rather than break), and those which have greater hardness : Inert Minerals: Inert Oxide Minerals: Cassiterite (tin oxide) Chromite (chromium oxide) Rutile (titanium oxide) Magnetite (iron oxide) Ilmenite (iron titanium oxide) Inert Silicate Minerals: Wolframite Zircon Maleable Minerals: Native Metals: Gold Platinum Bismuth Hard Minerals: Diamond Corundum Garnet
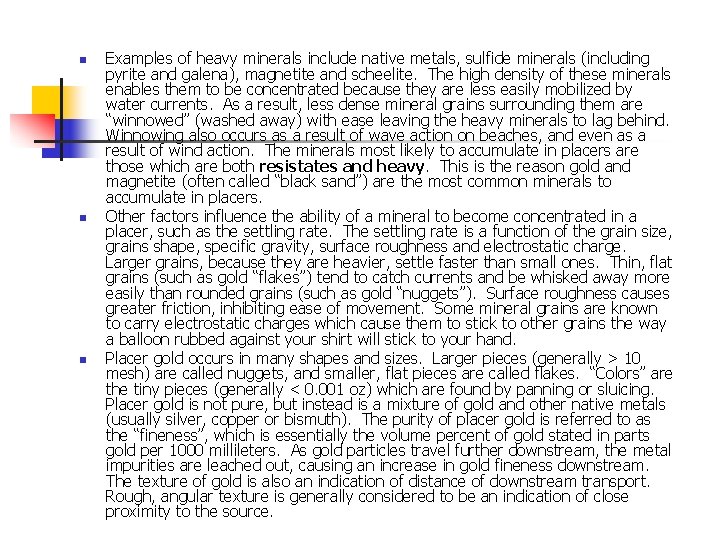
n n n Examples of heavy minerals include native metals, sulfide minerals (including pyrite and galena), magnetite and scheelite. The high density of these minerals enables them to be concentrated because they are less easily mobilized by water currents. As a result, less dense mineral grains surrounding them are “winnowed” (washed away) with ease leaving the heavy minerals to lag behind. Winnowing also occurs as a result of wave action on beaches, and even as a result of wind action. The minerals most likely to accumulate in placers are those which are both resistates and heavy. This is the reason gold and magnetite (often called “black sand”) are the most common minerals to accumulate in placers. Other factors influence the ability of a mineral to become concentrated in a placer, such as the settling rate. The settling rate is a function of the grain size, grains shape, specific gravity, surface roughness and electrostatic charge. Larger grains, because they are heavier, settle faster than small ones. Thin, flat grains (such as gold “flakes”) tend to catch currents and be whisked away more easily than rounded grains (such as gold “nuggets”). Surface roughness causes greater friction, inhibiting ease of movement. Some mineral grains are known to carry electrostatic charges which cause them to stick to other grains the way a balloon rubbed against your shirt will stick to your hand. Placer gold occurs in many shapes and sizes. Larger pieces (generally > 10 mesh) are called nuggets, and smaller, flat pieces are called flakes. “Colors” are the tiny pieces (generally < 0. 001 oz) which are found by panning or sluicing. Placer gold is not pure, but instead is a mixture of gold and other native metals (usually silver, copper or bismuth). The purity of placer gold is referred to as the “fineness”, which is essentially the volume percent of gold stated in parts gold per 1000 millileters. As gold particles travel further downstream, the metal impurities are leached out, causing an increase in gold fineness downstream. The texture of gold is also an indication of distance of downstream transport. Rough, angular texture is generally considered to be an indication of close proximity to the source.
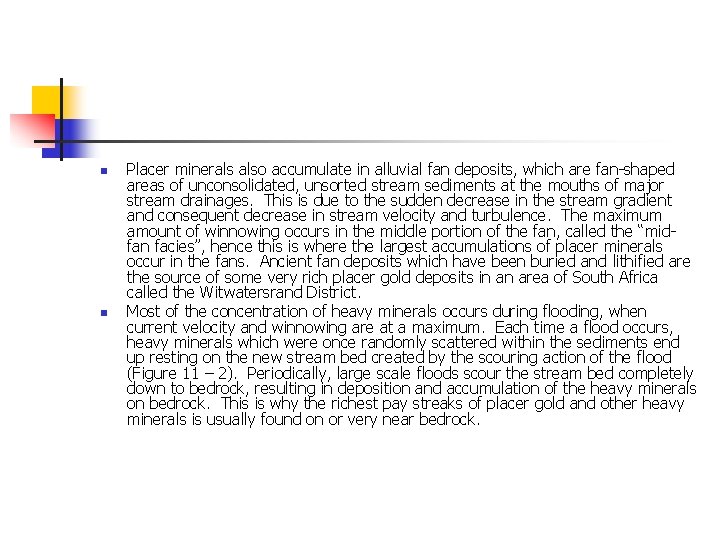
n n Placer minerals also accumulate in alluvial fan deposits, which are fan-shaped areas of unconsolidated, unsorted stream sediments at the mouths of major stream drainages. This is due to the sudden decrease in the stream gradient and consequent decrease in stream velocity and turbulence. The maximum amount of winnowing occurs in the middle portion of the fan, called the “midfan facies”, hence this is where the largest accumulations of placer minerals occur in the fans. Ancient fan deposits which have been buried and lithified are the source of some very rich placer gold deposits in an area of South Africa called the Witwatersrand District. Most of the concentration of heavy minerals occurs during flooding, when current velocity and winnowing are at a maximum. Each time a flood occurs, heavy minerals which were once randomly scattered within the sediments end up resting on the new stream bed created by the scouring action of the flood (Figure 11 – 2). Periodically, large scale floods scour the stream bed completely down to bedrock, resulting in deposition and accumulation of the heavy minerals on bedrock. This is why the richest pay streaks of placer gold and other heavy minerals is usually found on or very near bedrock.
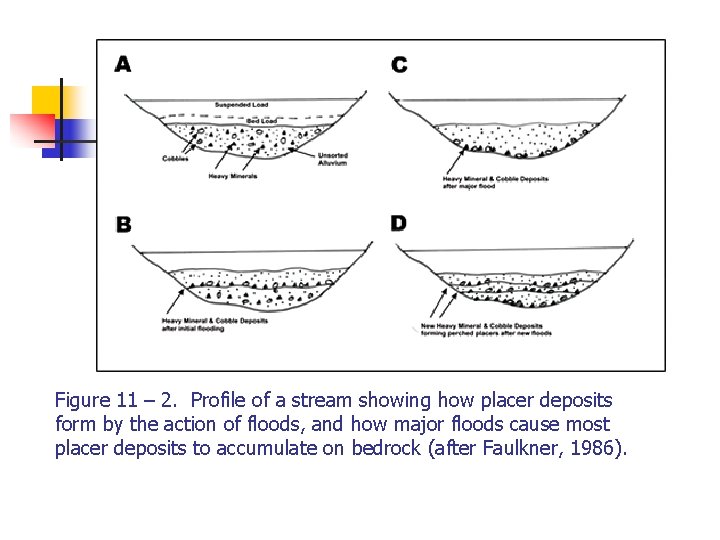
Figure 11 – 2. Profile of a stream showing how placer deposits form by the action of floods, and how major floods cause most placer deposits to accumulate on bedrock (after Faulkner, 1986).
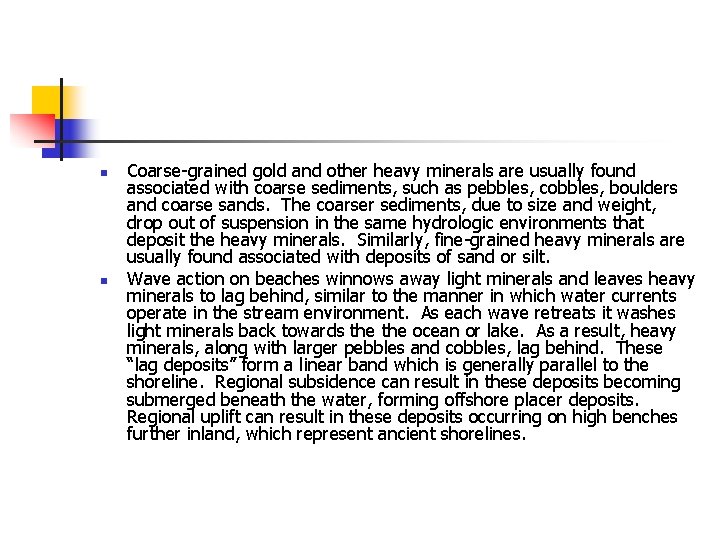
n n Coarse-grained gold and other heavy minerals are usually found associated with coarse sediments, such as pebbles, cobbles, boulders and coarse sands. The coarser sediments, due to size and weight, drop out of suspension in the same hydrologic environments that deposit the heavy minerals. Similarly, fine-grained heavy minerals are usually found associated with deposits of sand or silt. Wave action on beaches winnows away light minerals and leaves heavy minerals to lag behind, similar to the manner in which water currents operate in the stream environment. As each wave retreats it washes light minerals back towards the ocean or lake. As a result, heavy minerals, along with larger pebbles and cobbles, lag behind. These “lag deposits” form a linear band which is generally parallel to the shoreline. Regional subsidence can result in these deposits becoming submerged beneath the water, forming offshore placer deposits. Regional uplift can result in these deposits occurring on high benches further inland, which represent ancient shorelines.
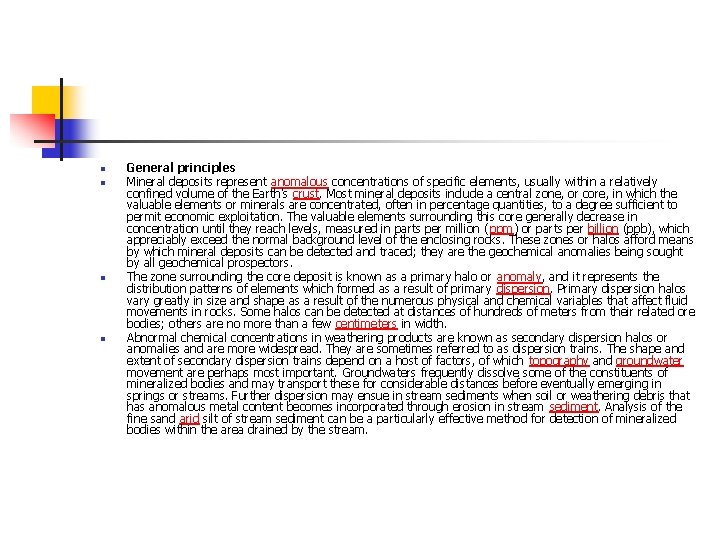
n n General principles Mineral deposits represent anomalous concentrations of specific elements, usually within a relatively confined volume of the Earth's crust. Most mineral deposits include a central zone, or core, in which the valuable elements or minerals are concentrated, often in percentage quantities, to a degree sufficient to permit economic exploitation. The valuable elements surrounding this core generally decrease in concentration until they reach levels, measured in parts per million ( ppm) or parts per billion (ppb), which appreciably exceed the normal background level of the enclosing rocks. These zones or halos afford means by which mineral deposits can be detected and traced; they are the geochemical anomalies being sought by all geochemical prospectors. The zone surrounding the core deposit is known as a primary halo or anomaly, and it represents the distribution patterns of elements which formed as a result of primary dispersion. Primary dispersion halos vary greatly in size and shape as a result of the numerous physical and chemical variables that affect fluid movements in rocks. Some halos can be detected at distances of hundreds of meters from their related ore bodies; others are no more than a few centimeters in width. Abnormal chemical concentrations in weathering products are known as secondary dispersion halos or anomalies and are more widespread. They are sometimes referred to as dispersion trains. The shape and extent of secondary dispersion trains depend on a host of factors, of which topography and groundwater movement are perhaps most important. Groundwaters frequently dissolve some of the constituents of mineralized bodies and may transport these for considerable distances before eventually emerging in springs or streams. Further dispersion may ensue in stream sediments when soil or weathering debris that has anomalous metal content becomes incorporated through erosion in stream sediment. Analysis of the fine sand arid silt of stream sediment can be a particularly effective method for detection of mineralized bodies within the area drained by the stream.
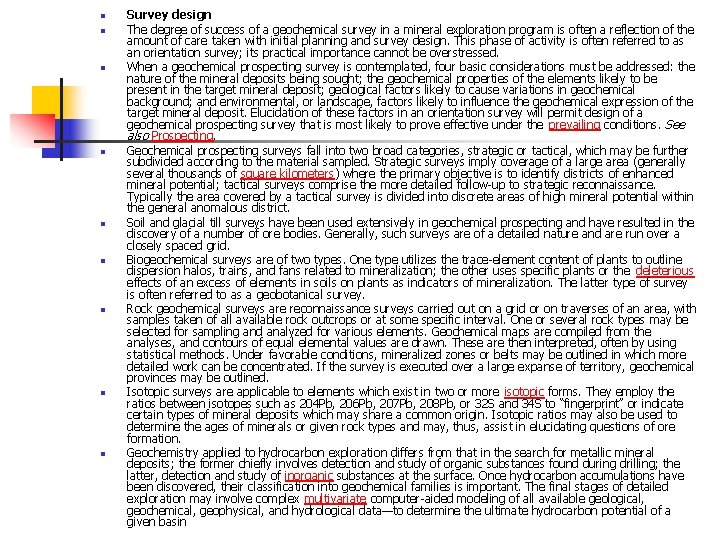
n n n n n Survey design The degree of success of a geochemical survey in a mineral exploration program is often a reflection of the amount of care taken with initial planning and survey design. This phase of activity is often referred to as an orientation survey; its practical importance cannot be overstressed. When a geochemical prospecting survey is contemplated, four basic considerations must be addressed: the nature of the mineral deposits being sought; the geochemical properties of the elements likely to be present in the target mineral deposit; geological factors likely to cause variations in geochemical background; and environmental, or landscape, factors likely to influence the geochemical expression of the target mineral deposit. Elucidation of these factors in an orientation survey will permit design of a geochemical prospecting survey that is most likely to prove effective under the prevailing conditions. See also Prospecting. Geochemical prospecting surveys fall into two broad categories, strategic or tactical, which may be further subdivided according to the material sampled. Strategic surveys imply coverage of a large area (generally several thousands of square kilometers) where the primary objective is to identify districts of enhanced mineral potential; tactical surveys comprise the more detailed follow-up to strategic reconnaissance. Typically the area covered by a tactical survey is divided into discrete areas of high mineral potential within the general anomalous district. Soil and glacial till surveys have been used extensively in geochemical prospecting and have resulted in the discovery of a number of ore bodies. Generally, such surveys are of a detailed nature and are run over a closely spaced grid. Biogeochemical surveys are of two types. One type utilizes the trace-element content of plants to outline dispersion halos, trains, and fans related to mineralization; the other uses specific plants or the deleterious effects of an excess of elements in soils on plants as indicators of mineralization. The latter type of survey is often referred to as a geobotanical survey. Rock geochemical surveys are reconnaissance surveys carried out on a grid or on traverses of an area, with samples taken of all available rock outcrops or at some specific interval. One or several rock types may be selected for sampling and analyzed for various elements. Geochemical maps are compiled from the analyses, and contours of equal elemental values are drawn. These are then interpreted, often by using statistical methods. Under favorable conditions, mineralized zones or belts may be outlined in which more detailed work can be concentrated. If the survey is executed over a large expanse of territory, geochemical provinces may be outlined. Isotopic surveys are applicable to elements which exist in two or more isotopic forms. They employ the ratios between isotopes such as 204 Pb, 206 Pb, 207 Pb, 208 Pb, or 32 S and 34 S to “fingerprint” or indicate certain types of mineral deposits which may share a common origin. Isotopic ratios may also be used to determine the ages of minerals or given rock types and may, thus, assist in elucidating questions of ore formation. Geochemistry applied to hydrocarbon exploration differs from that in the search for metallic mineral deposits; the former chiefly involves detection and study of organic substances found during drilling; the latter, detection and study of inorganic substances at the surface. Once hydrocarbon accumulations have been discovered, their classification into geochemical families is important. The final stages of detailed exploration may involve complex multivariate computer-aided modeling of all available geological, geochemical, geophysical, and hydrological data—to determine the ultimate hydrocarbon potential of a given basin
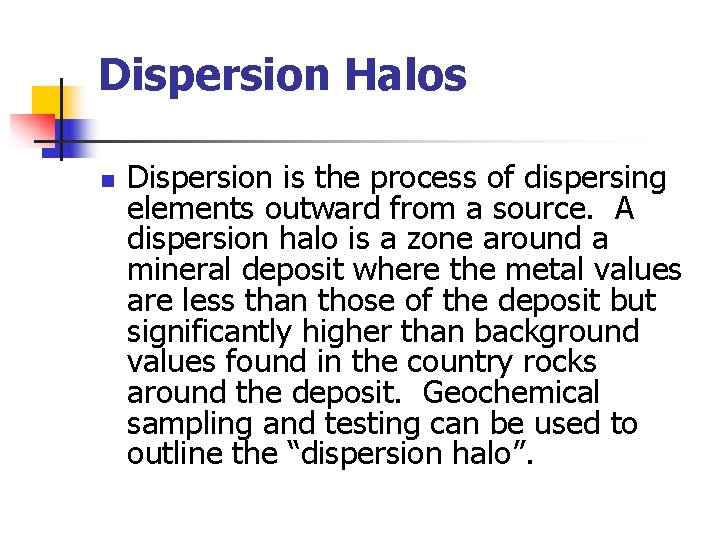
Dispersion Halos n Dispersion is the process of dispersing elements outward from a source. A dispersion halo is a zone around a mineral deposit where the metal values are less than those of the deposit but significantly higher than background values found in the country rocks around the deposit. Geochemical sampling and testing can be used to outline the “dispersion halo”.
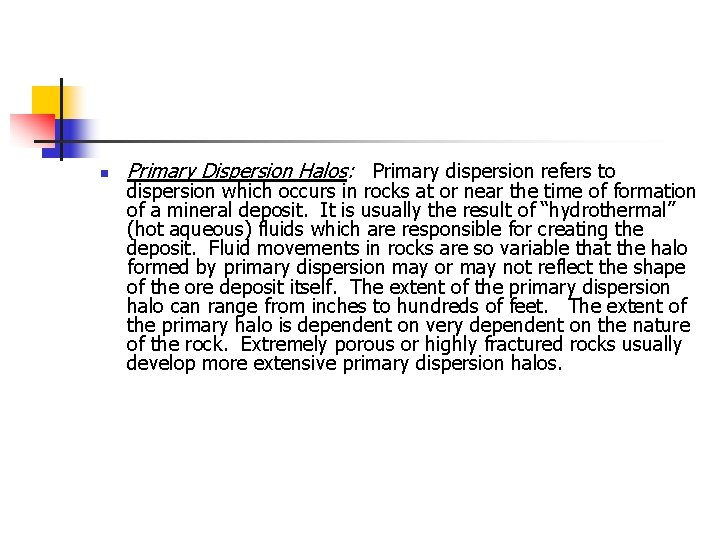
n Primary Dispersion Halos: Primary dispersion refers to dispersion which occurs in rocks at or near the time of formation of a mineral deposit. It is usually the result of “hydrothermal” (hot aqueous) fluids which are responsible for creating the deposit. Fluid movements in rocks are so variable that the halo formed by primary dispersion may or may not reflect the shape of the ore deposit itself. The extent of the primary dispersion halo can range from inches to hundreds of feet. The extent of the primary halo is dependent on very dependent on the nature of the rock. Extremely porous or highly fractured rocks usually develop more extensive primary dispersion halos.
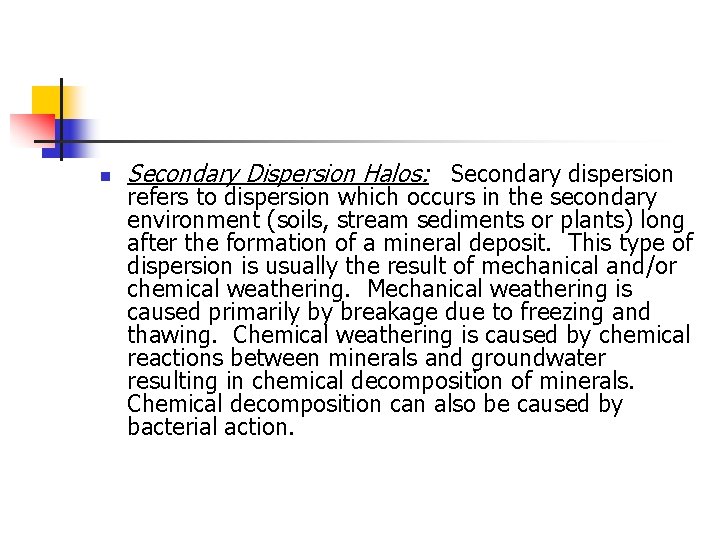
n Secondary Dispersion Halos: Secondary dispersion refers to dispersion which occurs in the secondary environment (soils, stream sediments or plants) long after the formation of a mineral deposit. This type of dispersion is usually the result of mechanical and/or chemical weathering. Mechanical weathering is caused primarily by breakage due to freezing and thawing. Chemical weathering is caused by chemical reactions between minerals and groundwater resulting in chemical decomposition of minerals. Chemical decomposition can also be caused by bacterial action.
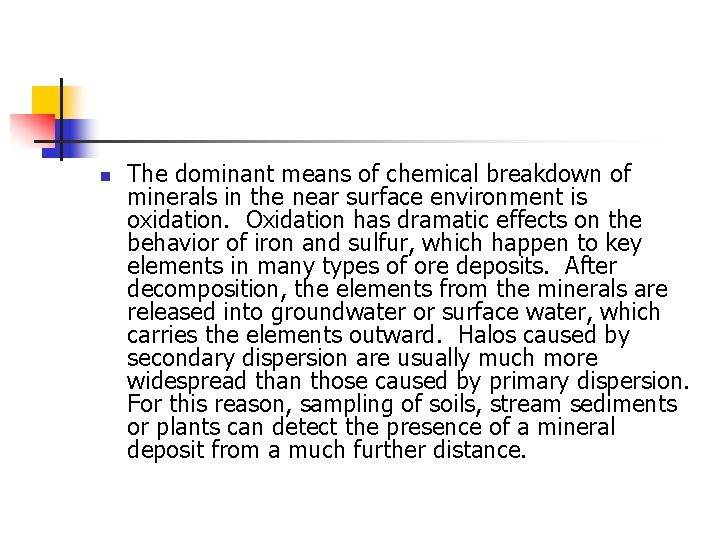
n The dominant means of chemical breakdown of minerals in the near surface environment is oxidation. Oxidation has dramatic effects on the behavior of iron and sulfur, which happen to key elements in many types of ore deposits. After decomposition, the elements from the minerals are released into groundwater or surface water, which carries the elements outward. Halos caused by secondary dispersion are usually much more widespread than those caused by primary dispersion. For this reason, sampling of soils, stream sediments or plants can detect the presence of a mineral deposit from a much further distance.
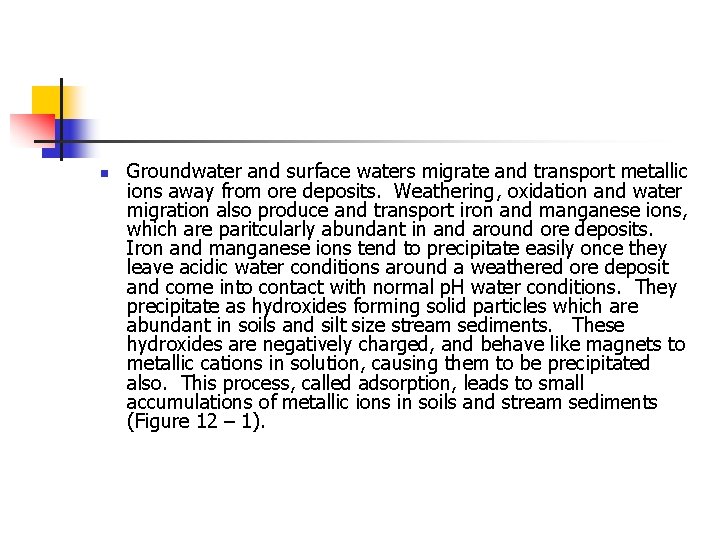
n Groundwater and surface waters migrate and transport metallic ions away from ore deposits. Weathering, oxidation and water migration also produce and transport iron and manganese ions, which are paritcularly abundant in and around ore deposits. Iron and manganese ions tend to precipitate easily once they leave acidic water conditions around a weathered ore deposit and come into contact with normal p. H water conditions. They precipitate as hydroxides forming solid particles which are abundant in soils and silt size stream sediments. These hydroxides are negatively charged, and behave like magnets to metallic cations in solution, causing them to be precipitated also. This process, called adsorption, leads to small accumulations of metallic ions in soils and stream sediments (Figure 12 – 1).
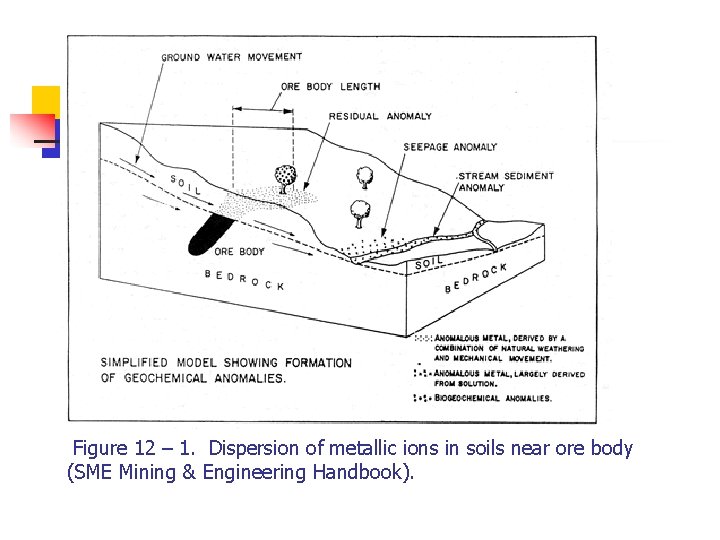
Figure 12 – 1. Dispersion of metallic ions in soils near ore body (SME Mining & Engineering Handbook).
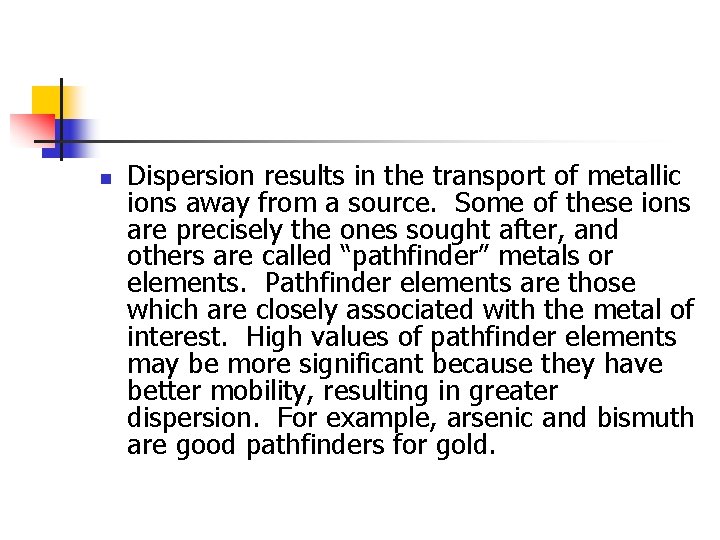
n Dispersion results in the transport of metallic ions away from a source. Some of these ions are precisely the ones sought after, and others are called “pathfinder” metals or elements. Pathfinder elements are those which are closely associated with the metal of interest. High values of pathfinder elements may be more significant because they have better mobility, resulting in greater dispersion. For example, arsenic and bismuth are good pathfinders for gold.
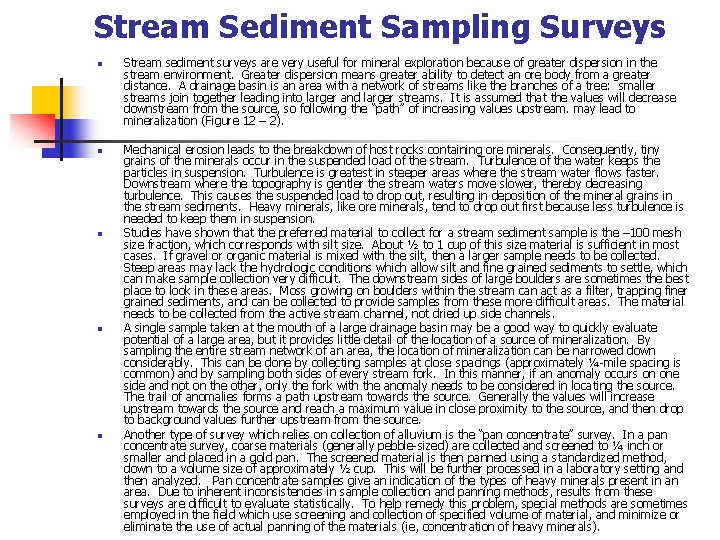
Stream Sediment Sampling Surveys n n n Stream sediment surveys are very useful for mineral exploration because of greater dispersion in the stream environment. Greater dispersion means greater ability to detect an ore body from a greater distance. A drainage basin is an area with a network of streams like the branches of a tree: smaller streams join together leading into larger and larger streams. It is assumed that the values will decrease downstream from the source, so following the “path” of increasing values upstream. may lead to mineralization (Figure 12 – 2). Mechanical erosion leads to the breakdown of host rocks containing ore minerals. Consequently, tiny grains of the minerals occur in the suspended load of the stream. Turbulence of the water keeps the particles in suspension. Turbulence is greatest in steeper areas where the stream water flows faster. Downstream where the topography is gentler the stream waters move slower, thereby decreasing turbulence. This causes the suspended load to drop out, resulting in deposition of the mineral grains in the stream sediments. Heavy minerals, like ore minerals, tend to drop out first because less turbulence is needed to keep them in suspension. Studies have shown that the preferred material to collect for a stream sediment sample is the – 100 mesh size fraction, which corresponds with silt size. About ½ to 1 cup of this size material is sufficient in most cases. If gravel or organic material is mixed with the silt, then a larger sample needs to be collected. Steep areas may lack the hydrologic conditions which allow silt and fine grained sediments to settle, which can make sample collection very difficult. The downstream sides of large boulders are sometimes the best place to look in these areas. Moss growing on boulders within the stream can act as a filter, trapping finer grained sediments, and can be collected to provide samples from these more difficult areas. The material needs to be collected from the active stream channel, not dried up side channels. A single sample taken at the mouth of a large drainage basin may be a good way to quickly evaluate potential of a large area, but it provides little detail of the location of a source of mineralization. By sampling the entire stream network of an area, the location of mineralization can be narrowed down considerably. This can be done by collecting samples at close spacings (approximately ¼-mile spacing is common) and by sampling both sides of every stream fork. In this manner, if an anomaly occurs on one side and not on the other, only the fork with the anomaly needs to be considered in locating the source. The trail of anomalies forms a path upstream towards the source. Generally the values will increase upstream towards the source and reach a maximum value in close proximity to the source, and then drop to background values further upstream from the source. Another type of survey which relies on collection of alluvium is the “pan concentrate” survey. In a pan concentrate survey, coarse materials (generally pebble-sized) are collected and screened to ¼ inch or smaller and placed in a gold pan. The screened material is then panned using a standardized method, down to a volume size of approximately ½ cup. This will be further processed in a laboratory setting and then analyzed. Pan concentrate samples give an indication of the types of heavy minerals present in an area. Due to inherent inconsistencies in sample collection and panning methods, results from these surveys are difficult to evaluate statistically. To help remedy this problem, special methods are sometimes employed in the field which use screening and collection of specified volume of material, and minimize or eliminate the use of actual panning of the materials (ie, concentration of heavy minerals).
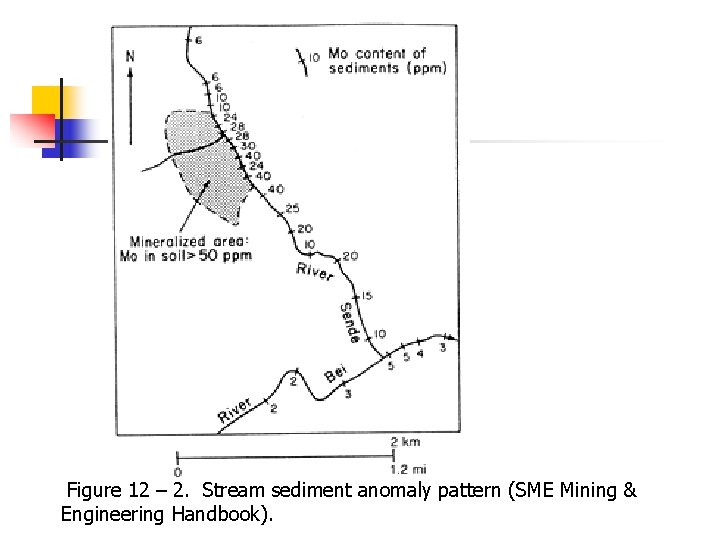
Figure 12 – 2. Stream sediment anomaly pattern (SME Mining & Engineering Handbook).
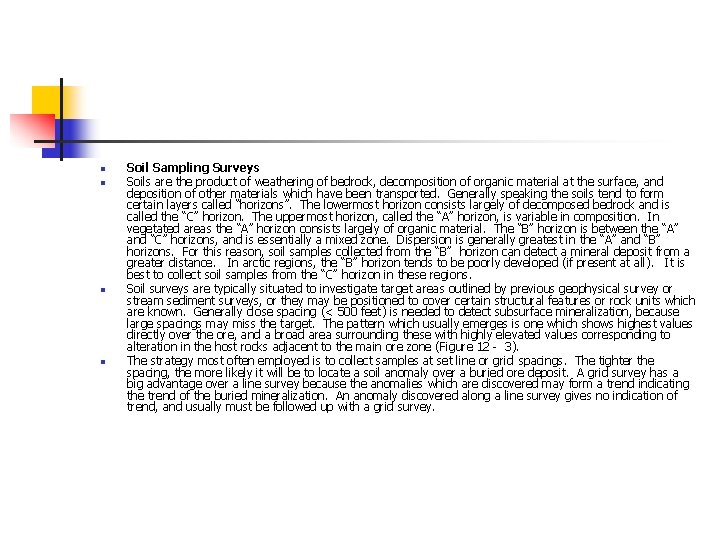
n n Soil Sampling Surveys Soils are the product of weathering of bedrock, decomposition of organic material at the surface, and deposition of other materials which have been transported. Generally speaking the soils tend to form certain layers called “horizons”. The lowermost horizon consists largely of decomposed bedrock and is called the “C” horizon. The uppermost horizon, called the “A” horizon, is variable in composition. In vegetated areas the “A” horizon consists largely of organic material. The “B” horizon is between the “A” and “C” horizons, and is essentially a mixed zone. Dispersion is generally greatest in the “A” and “B” horizons. For this reason, soil samples collected from the “B” horizon can detect a mineral deposit from a greater distance. In arctic regions, the “B” horizon tends to be poorly developed (if present at all). It is best to collect soil samples from the “C” horizon in these regions. Soil surveys are typically situated to investigate target areas outlined by previous geophysical survey or stream sediment surveys, or they may be positioned to cover certain structural features or rock units which are known. Generally close spacing (< 500 feet) is needed to detect subsurface mineralization, because large spacings may miss the target. The pattern which usually emerges is one which shows highest values directly over the ore, and a broad area surrounding these with highly elevated values corresponding to alteration in the host rocks adjacent to the main ore zone (Figure 12 - 3). The strategy most often employed is to collect samples at set line or grid spacings. The tighter the spacing, the more likely it will be to locate a soil anomaly over a buried ore deposit. A grid survey has a big advantage over a line survey because the anomalies which are discovered may form a trend indicating the trend of the buried mineralization. An anomaly discovered along a line survey gives no indication of trend, and usually must be followed up with a grid survey.
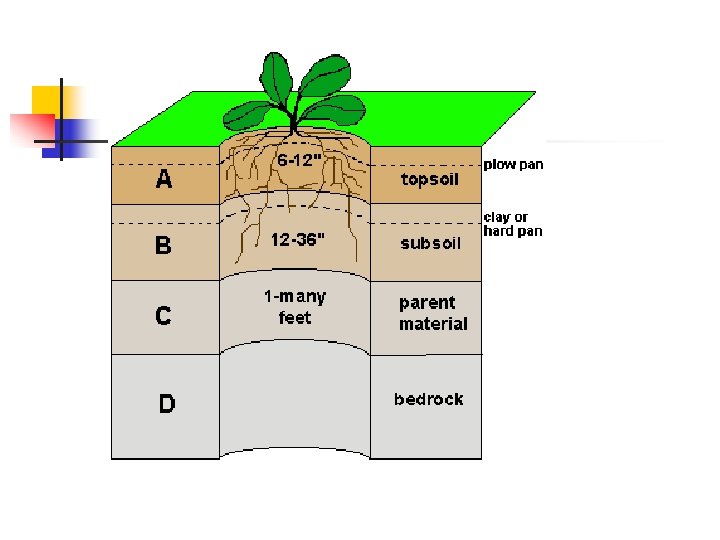
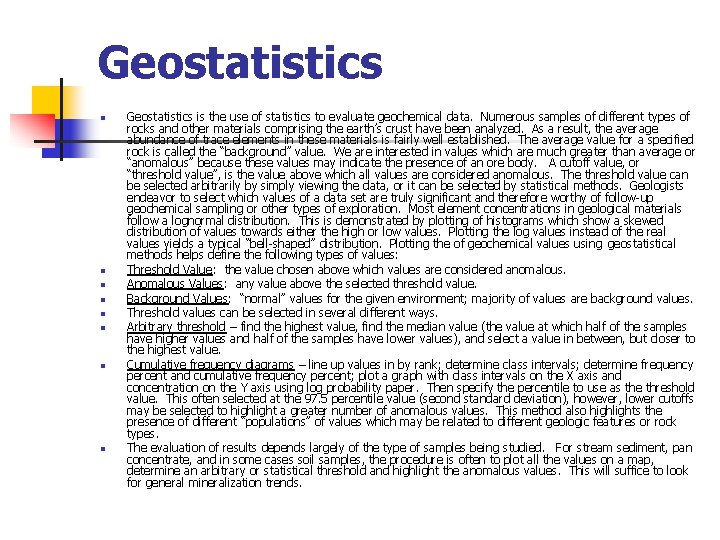
Geostatistics n n n n Geostatistics is the use of statistics to evaluate geochemical data. Numerous samples of different types of rocks and other materials comprising the earth’s crust have been analyzed. As a result, the average abundance of trace elements in these materials is fairly well established. The average value for a specified rock is called the “background” value. We are interested in values which are much greater than average or “anomalous” because these values may indicate the presence of an ore body. A cutoff value, or “threshold value”, is the value above which all values are considered anomalous. The threshold value can be selected arbitrarily by simply viewing the data, or it can be selected by statistical methods. Geologists endeavor to select which values of a data set are truly significant and therefore worthy of follow-up geochemical sampling or other types of exploration. Most element concentrations in geological materials follow a lognormal distribution. This is demonstrated by plotting of histograms which show a skewed distribution of values towards either the high or low values. Plotting the log values instead of the real values yields a typical “bell-shaped” distribution. Plotting the of geochemical values using geostatistical methods helps define the following types of values: Threshold Value: the value chosen above which values are considered anomalous. Anomalous Values: any value above the selected threshold value. Background Values: “normal” values for the given environment; majority of values are background values. Threshold values can be selected in several different ways. Arbitrary threshold – find the highest value, find the median value (the value at which half of the samples have higher values and half of the samples have lower values), and select a value in between, but closer to the highest value. Cumulative frequency diagrams – line up values in by rank; determine class intervals; determine frequency percent and cumulative frequency percent; plot a graph with class intervals on the X axis and concentration on the Y axis using log probability paper. Then specify the percentile to use as the threshold value. This often selected at the 97. 5 percentile value (second standard deviation), however, lower cutoffs may be selected to highlight a greater number of anomalous values. This method also highlights the presence of different “populations” of values which may be related to different geologic features or rock types. The evaluation of results depends largely of the type of samples being studied. For stream sediment, pan concentrate, and in some cases soil samples, the procedure is often to plot all the values on a map, determine an arbitrary or statistical threshold and highlight the anomalous values. This will suffice to look for general mineralization trends.
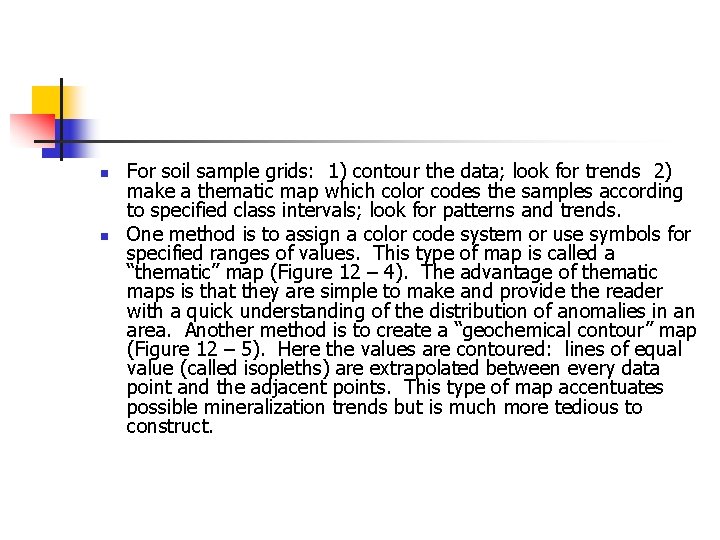
n n For soil sample grids: 1) contour the data; look for trends 2) make a thematic map which color codes the samples according to specified class intervals; look for patterns and trends. One method is to assign a color code system or use symbols for specified ranges of values. This type of map is called a “thematic” map (Figure 12 – 4). The advantage of thematic maps is that they are simple to make and provide the reader with a quick understanding of the distribution of anomalies in an area. Another method is to create a “geochemical contour” map (Figure 12 – 5). Here the values are contoured: lines of equal value (called isopleths) are extrapolated between every data point and the adjacent points. This type of map accentuates possible mineralization trends but is much more tedious to construct.
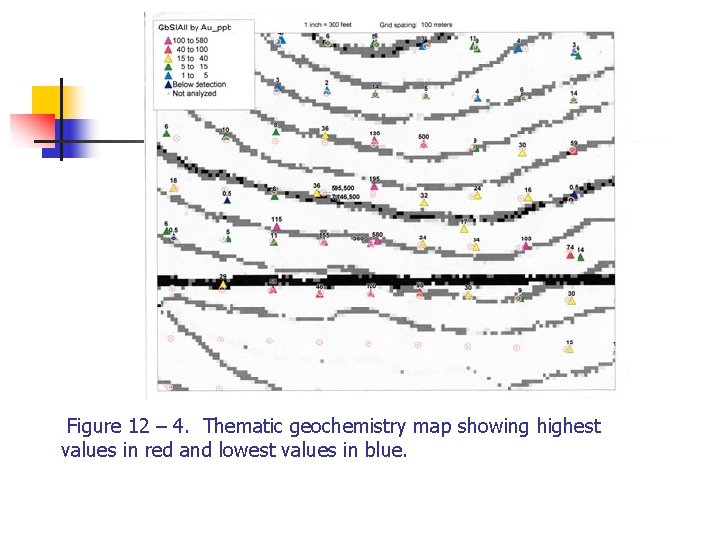
Figure 12 – 4. Thematic geochemistry map showing highest values in red and lowest values in blue.
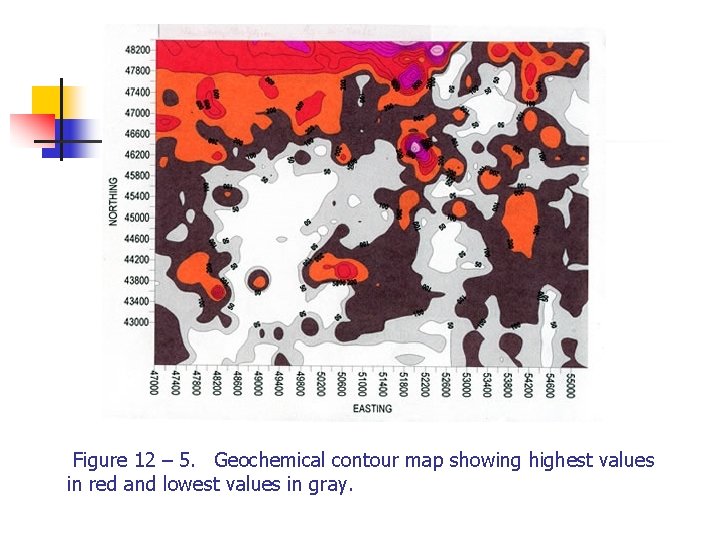
Figure 12 – 5. Geochemical contour map showing highest values in red and lowest values in gray.
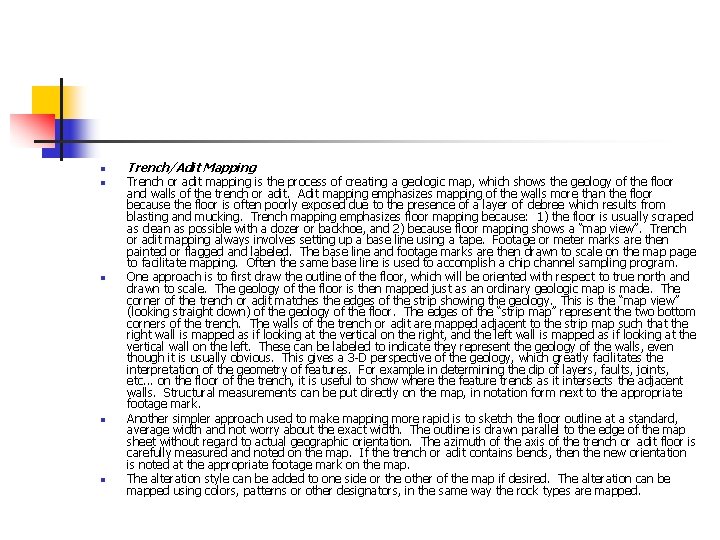
n n n Trench/Adit Mapping Trench or adit mapping is the process of creating a geologic map, which shows the geology of the floor and walls of the trench or adit. Adit mapping emphasizes mapping of the walls more than the floor because the floor is often poorly exposed due to the presence of a layer of debree which results from blasting and mucking. Trench mapping emphasizes floor mapping because: 1) the floor is usually scraped as clean as possible with a dozer or backhoe, and 2) because floor mapping shows a “map view”. Trench or adit mapping always involves setting up a base line using a tape. Footage or meter marks are then painted or flagged and labeled. The base line and footage marks are then drawn to scale on the map page to facilitate mapping. Often the same base line is used to accomplish a chip channel sampling program. One approach is to first draw the outline of the floor, which will be oriented with respect to true north and drawn to scale. The geology of the floor is then mapped just as an ordinary geologic map is made. The corner of the trench or adit matches the edges of the strip showing the geology. This is the “map view” (looking straight down) of the geology of the floor. The edges of the “strip map” represent the two bottom corners of the trench. The walls of the trench or adit are mapped adjacent to the strip map such that the right wall is mapped as if looking at the vertical on the right, and the left wall is mapped as if looking at the vertical wall on the left. These can be labeled to indicate they represent the geology of the walls, even though it is usually obvious. This gives a 3 -D perspective of the geology, which greatly facilitates the interpretation of the geometry of features. For example in determining the dip of layers, faults, joints, etc. . . on the floor of the trench, it is useful to show where the feature trends as it intersects the adjacent walls. Structural measurements can be put directly on the map, in notation form next to the appropriate footage mark. Another simpler approach used to make mapping more rapid is to sketch the floor outline at a standard, average width and not worry about the exact width. The outline is drawn parallel to the edge of the map sheet without regard to actual geographic orientation. The azimuth of the axis of the trench or adit floor is carefully measured and noted on the map. If the trench or adit contains bends, then the new orientation is noted at the appropriate footage mark on the map. The alteration style can be added to one side or the other of the map if desired. The alteration can be mapped using colors, patterns or other designators, in the same way the rock types are mapped.
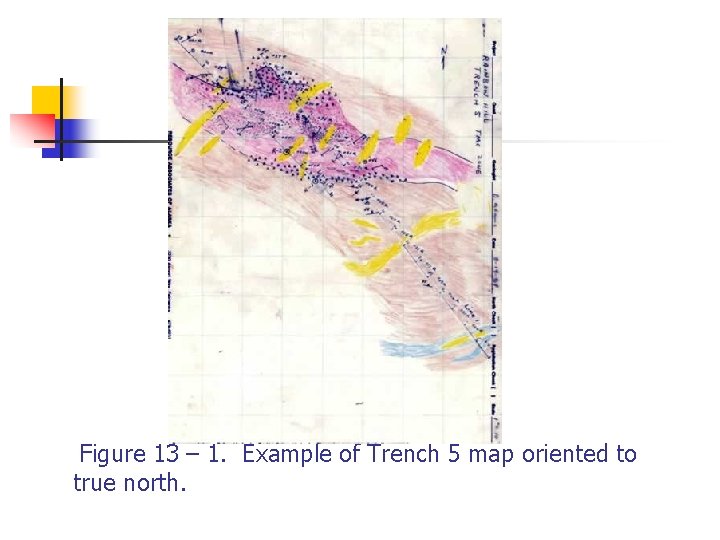
Figure 13 – 1. Example of Trench 5 map oriented to true north.
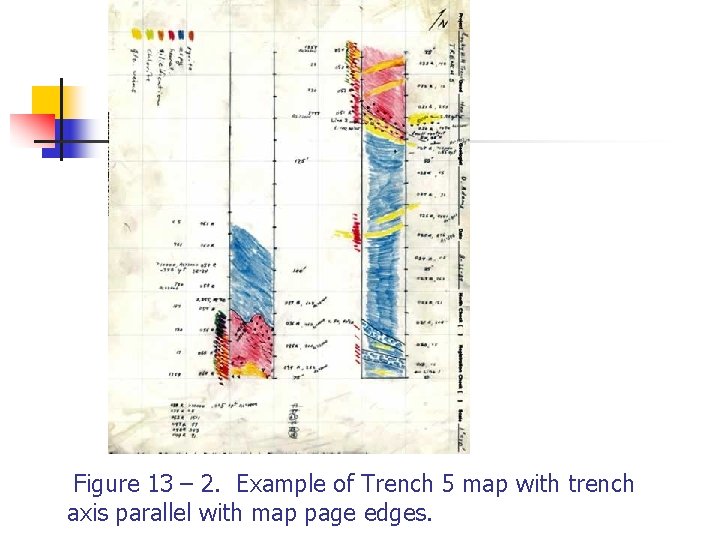
Figure 13 – 2. Example of Trench 5 map with trench axis parallel with map page edges.
Primary and secondary geochemical dispersion
Ahmed muhudiin ahmed
Practice geochemical cycles answer key
Ali madani md
Surah 14
Ali ahmed is a mathematics professor who tries to involve
Shirkat ul amwal
Ali is taller than ahmed
Differentiate between geological and accelerated erosion
Whats a contour map
Sedimentary rocks record past geological events and ____.
Plate tectonics vs continental drift
Geographical influence of roman architecture
Istvan van tuyl
Dharma wijewickreme
Geological map sweden
Us geological survey floating pan
Uniformly dipping beds
Geological map interpretation
Geological processes
Picture of relative dating
Graphic organizer geologic time scale
Rubric for designing geological time scale
Geological disaster example
Geological events
How do geological processes affect natural selection
The geological time scale
What do geological engineers invent
Geological
Geological fault
Divergent boundary geological features
Geological time scale with events
Tertiary period meaning
Geological time scale with events
Geological time scale
Geologic time
Makki surah
Ideology of pakistan by sir syed ahmed khan
Dr ahmed essop
Hotel classifications
Atieh madani
Surah rahman madani
Masyarakat madani dan kesejahteraan umat
Budaya demokrasi menuju masyarakat madani
Pt prima pangan madani
Pengertian istikomah
Ramtin madani
Aligarh movement
Uv.khayyam
Examples of inductive method
When the united indian patriotic association was set up
Ahmed salman rushdie
Ahmed salemi
Humbaracı ahmed paşa
Dr mohammed ahmed
Saad ahmed javed
Ahmed mustapha
Is an extended erp module
Dr mohammed ahmed
Ahmed helmy uf
Dr fawad randhawa
Anna molka ahmed artworks
Amina ahmed md
Aida ahmed
Ibrahim sammour
Kulsum ahmed
Ahmed miree
Shozab ahmed
Ahmed bawany academy school & college
Glutamate oxaloacetate transaminase test
Hezârfen ahmed çelebi meslek
Dr tahmeed ahmed
Chikhaoui ahmed
Shanzeh ahmed houston
Cse buet
Ahmed badr md
Ahmed zarouf
Dr ahmed hassan
Ihsan institute
Early life of sir syed ahmed khan
Bushra hasan
Dr. ahmed akl
Jasmine ahmed
Ahmed bououd
Ahmed helmy uf
Seyyid ahmed han
Dewan tanvir ahmed
Minterm
Iti 1100
Prevention of scabies ppt
Mohammed ashfaq ahmed
Dr. tamgid ahmed chowdhury
Manufacturing strategies
Paroxysmal nocturnal dyspnea pathophysiology
Dr fayyaz ahmed
Ahmed abu raghif
Dr.saeed ahmed
Adeela shafi