Commissioning experience of JPARC RCS Hiroyuki Harada JPARC
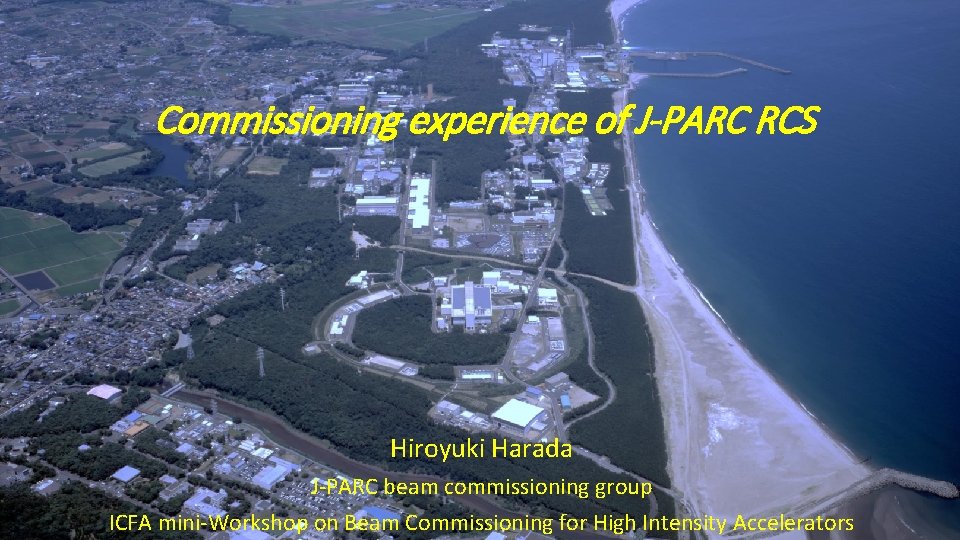
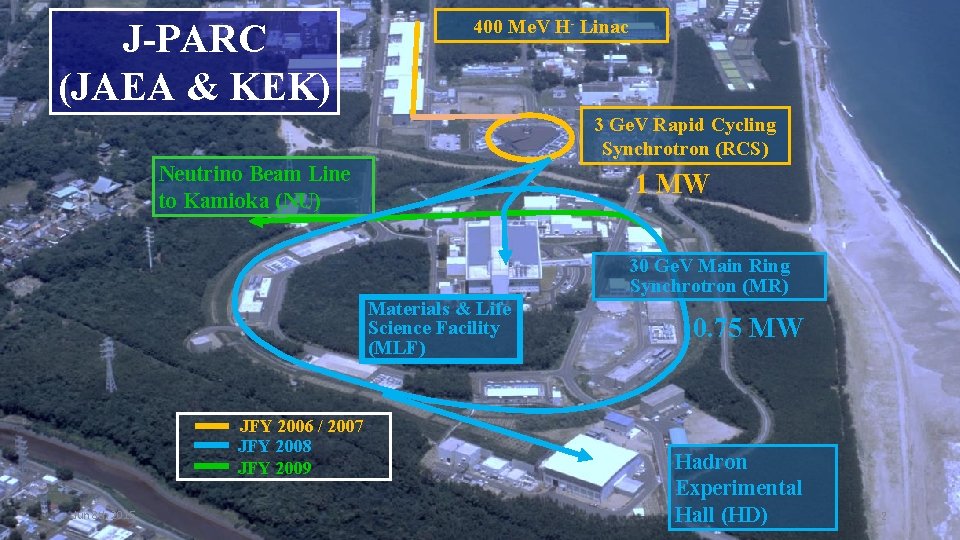
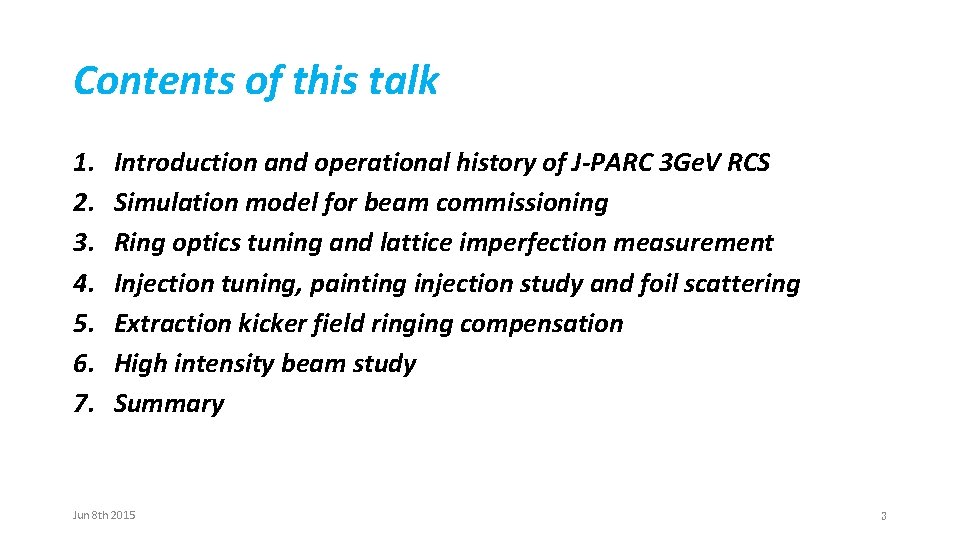
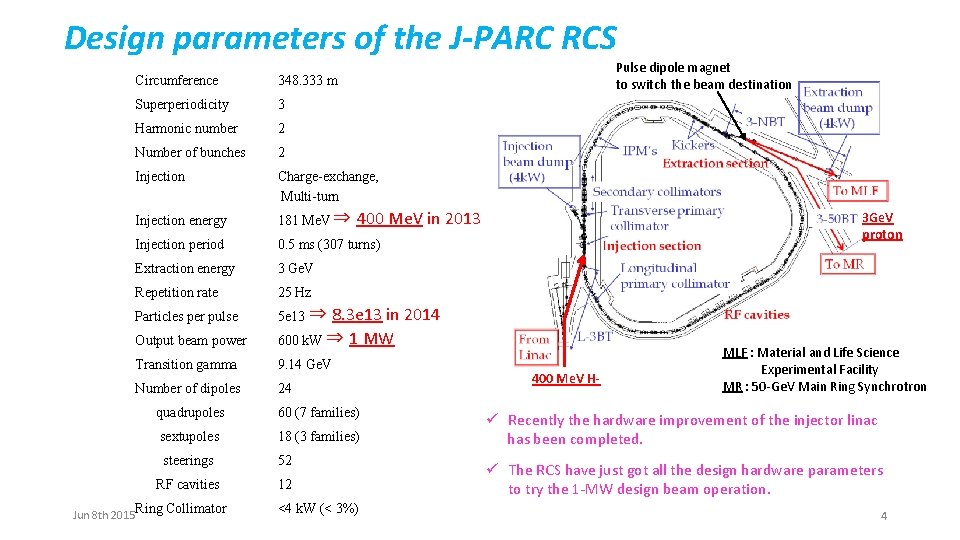
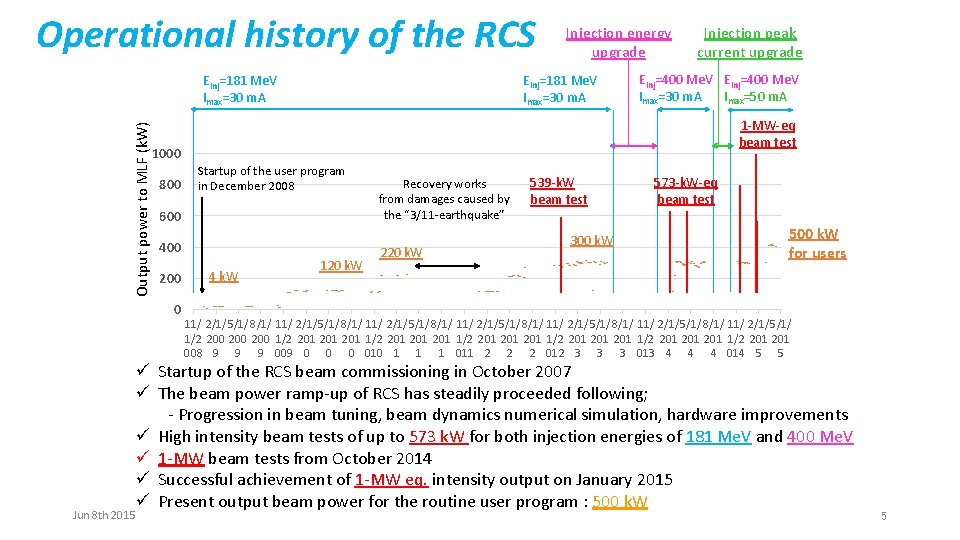
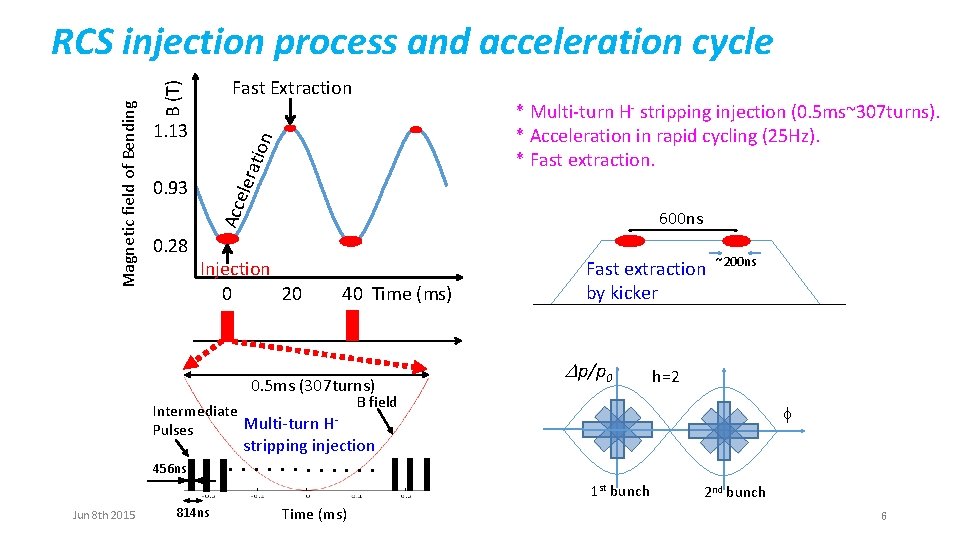
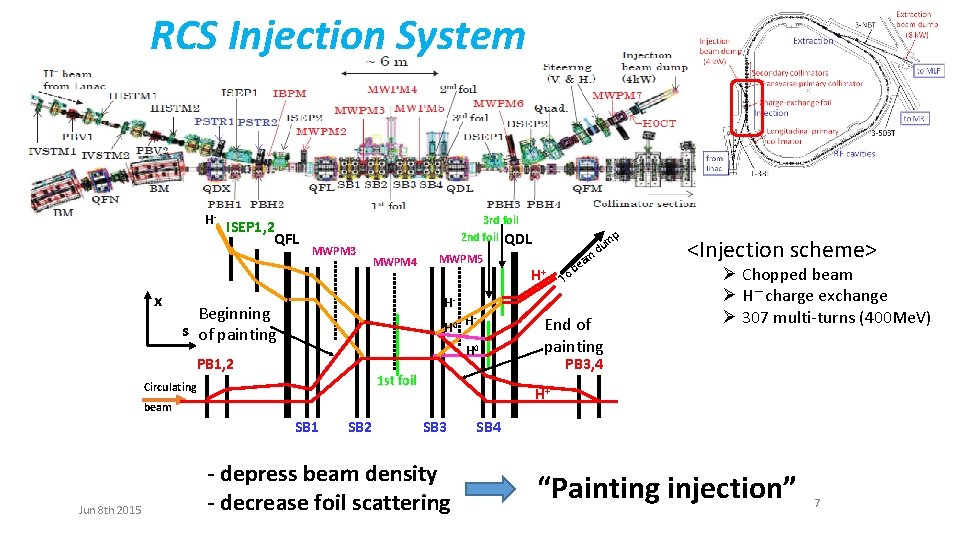
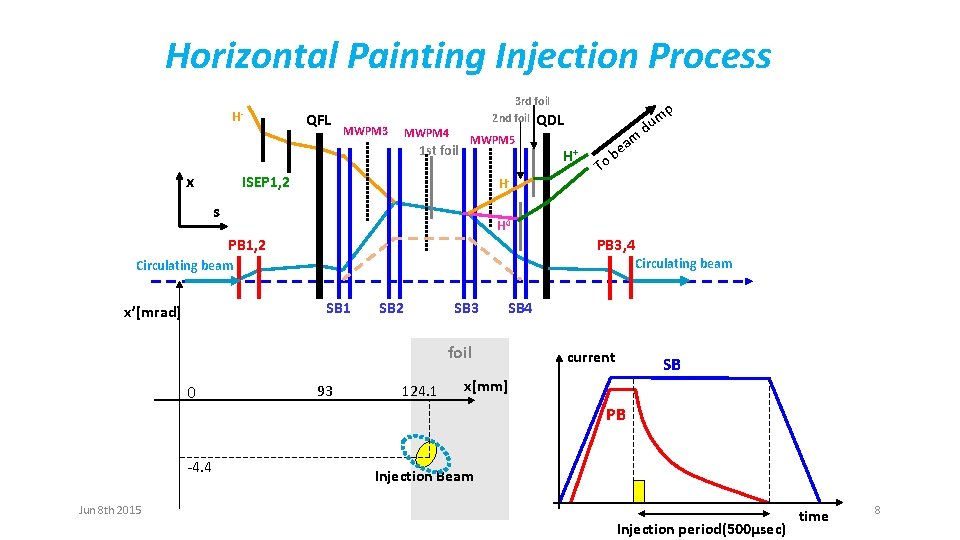
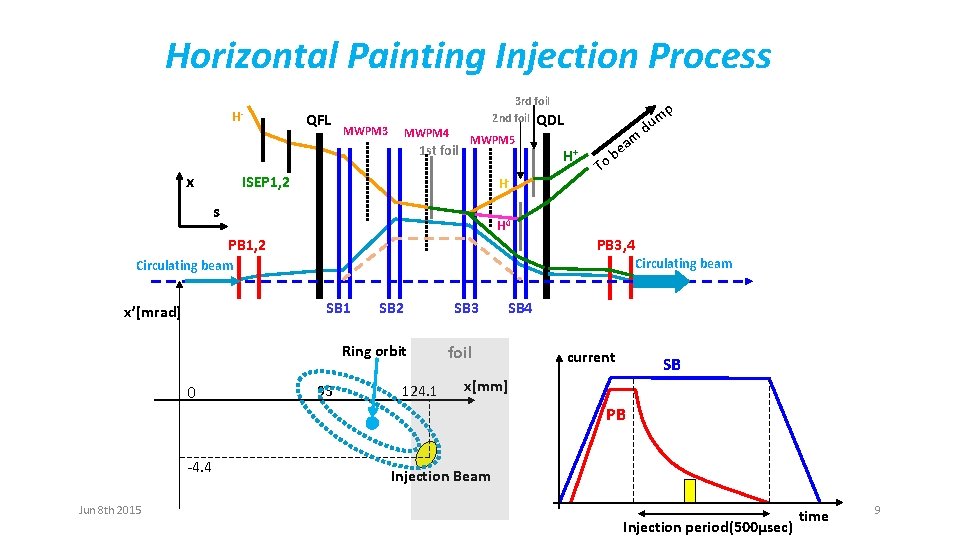
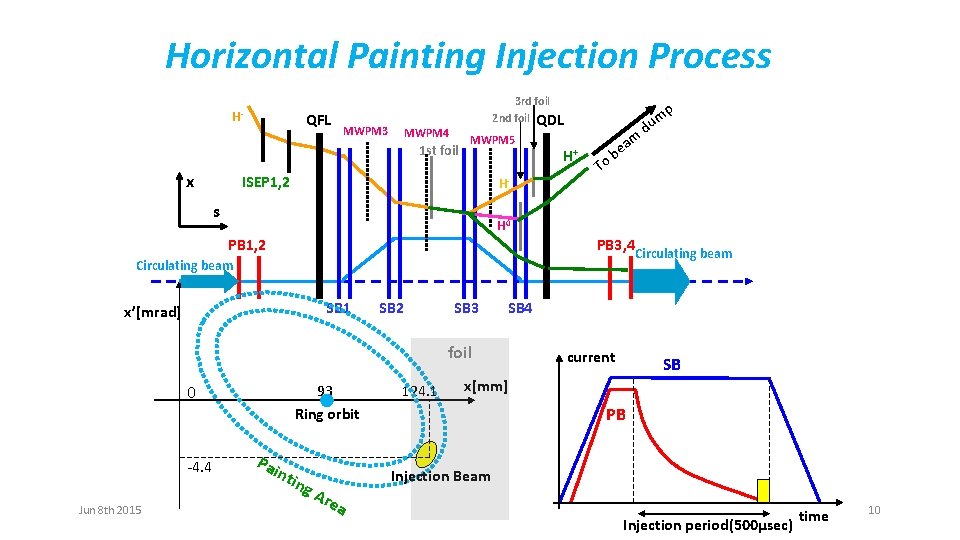
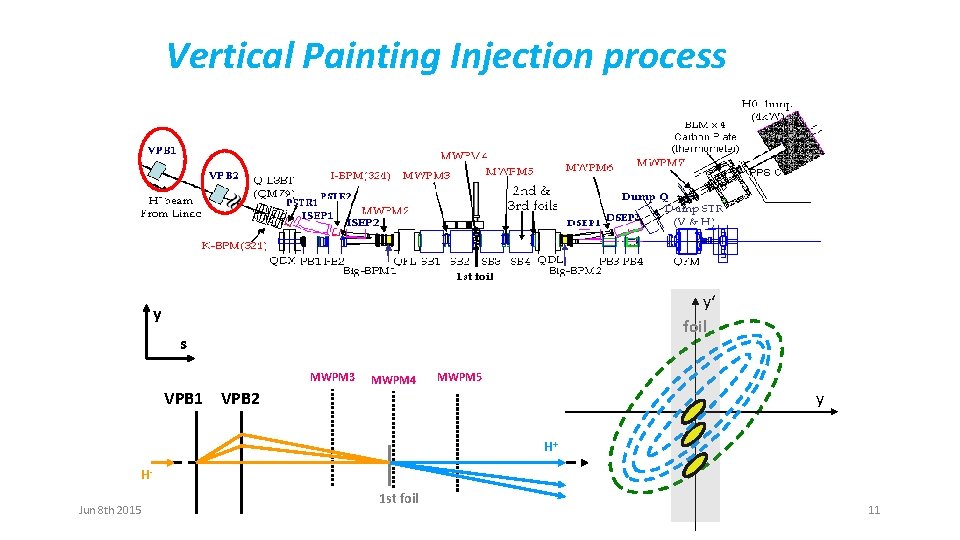
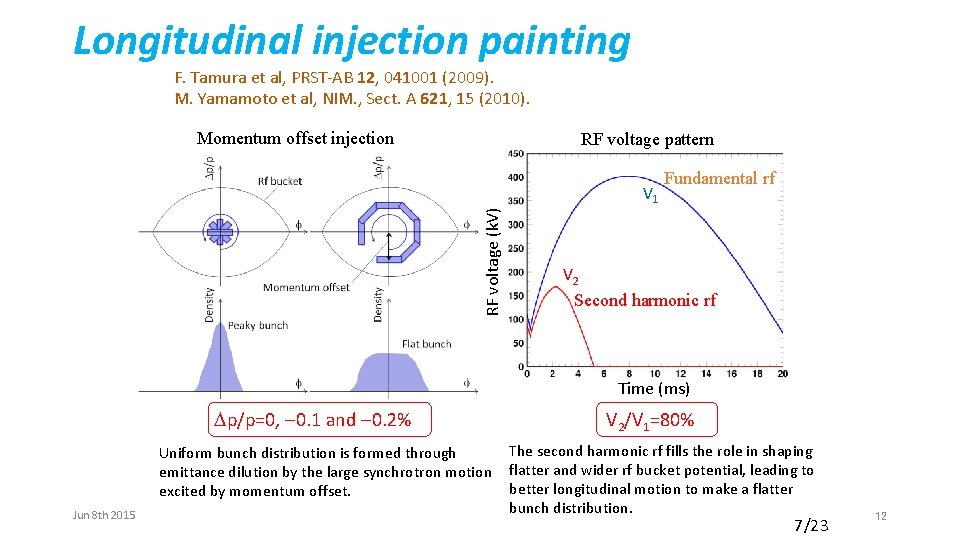
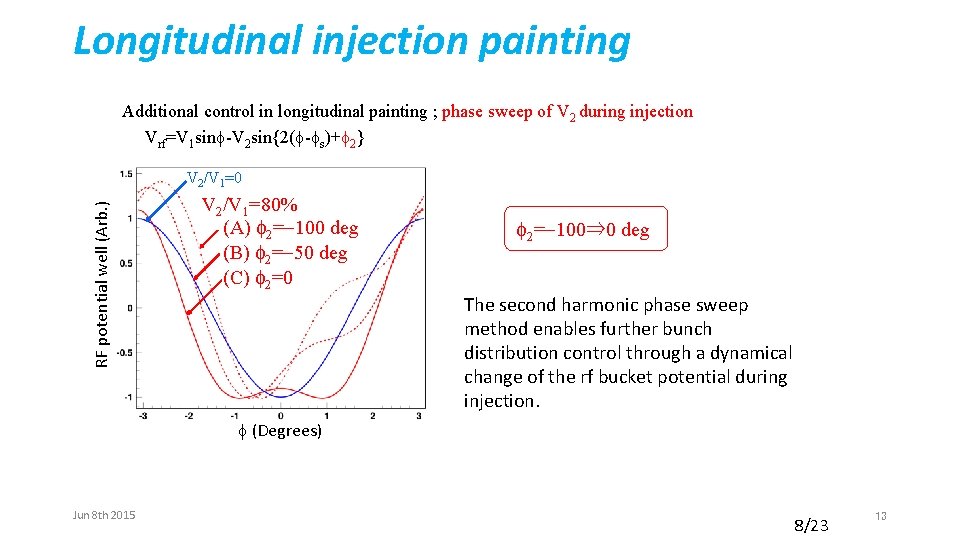
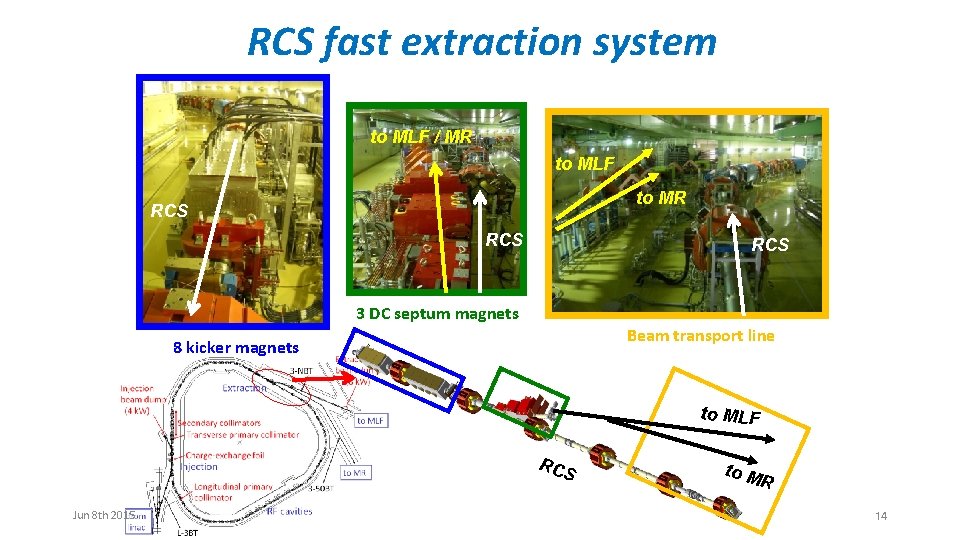
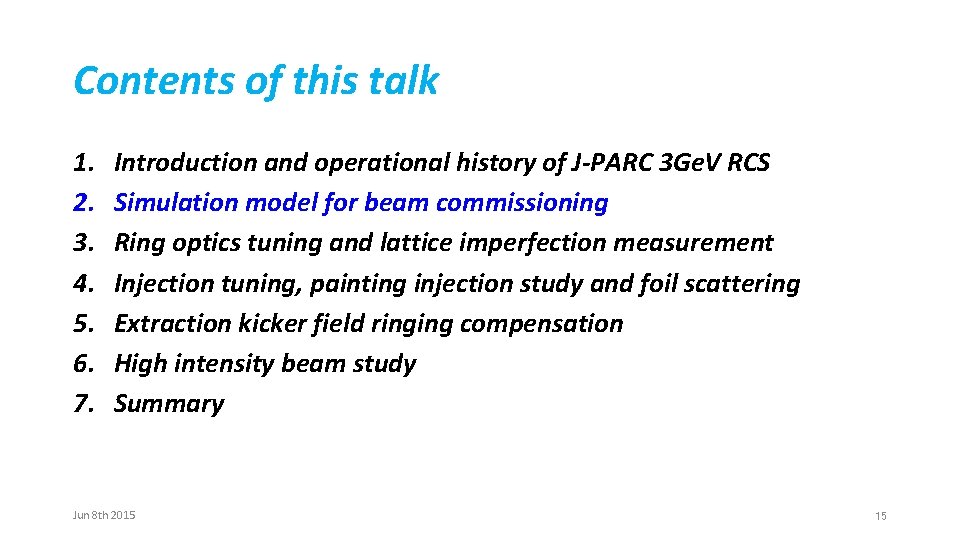
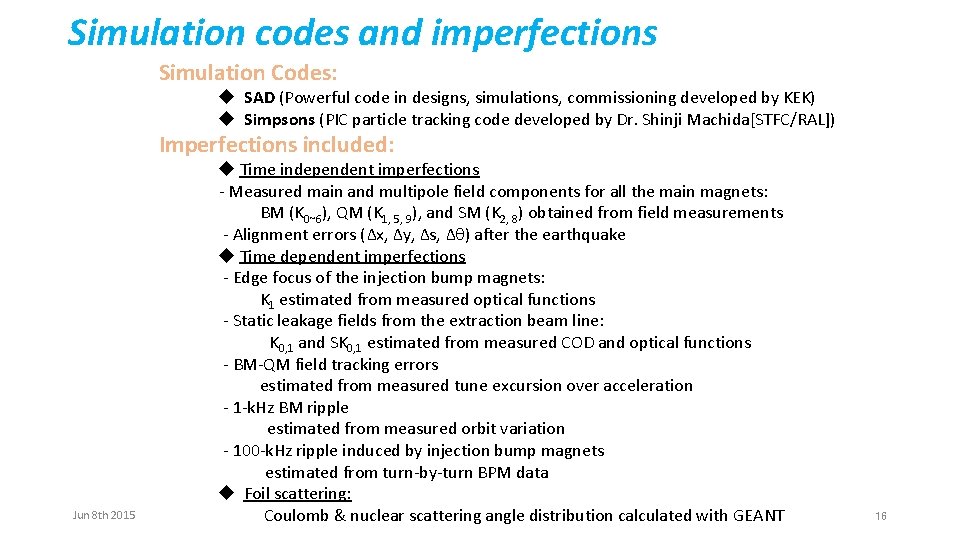
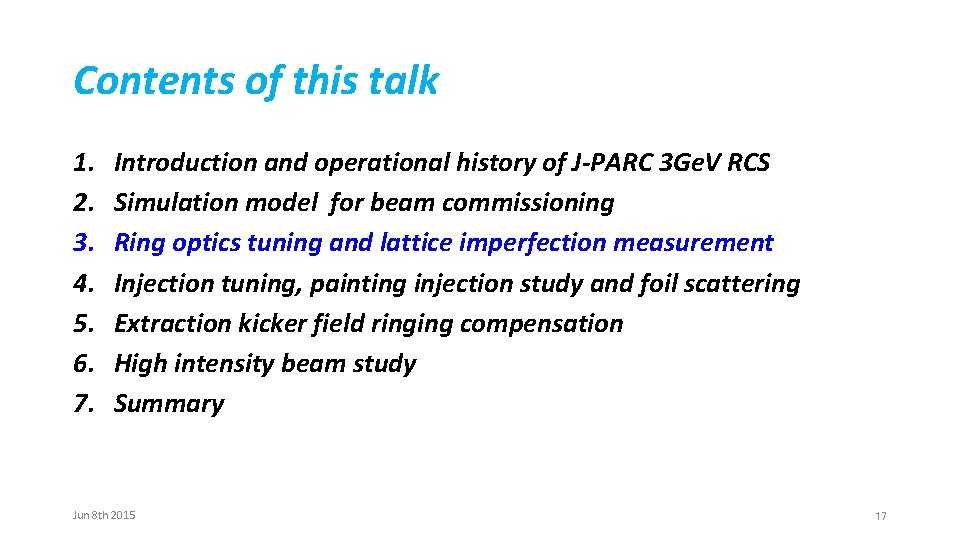
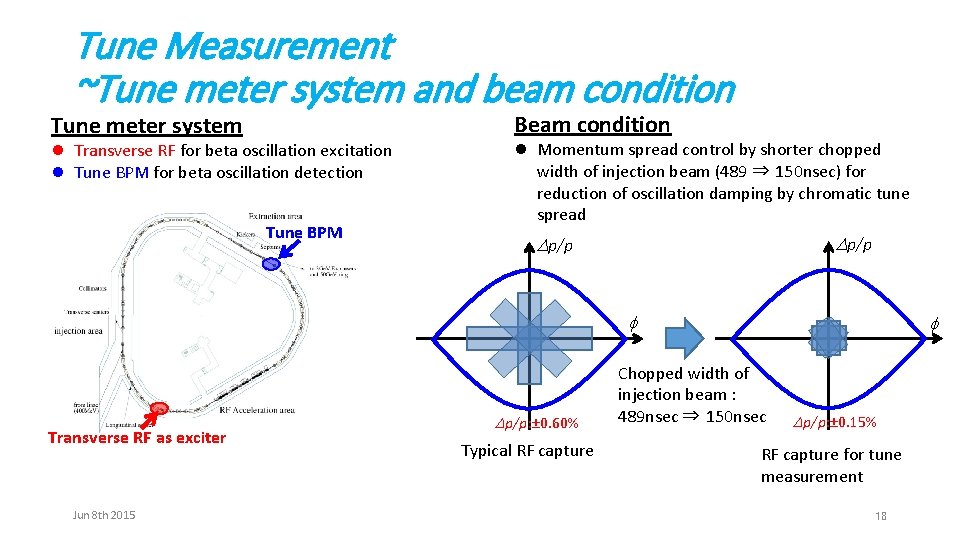
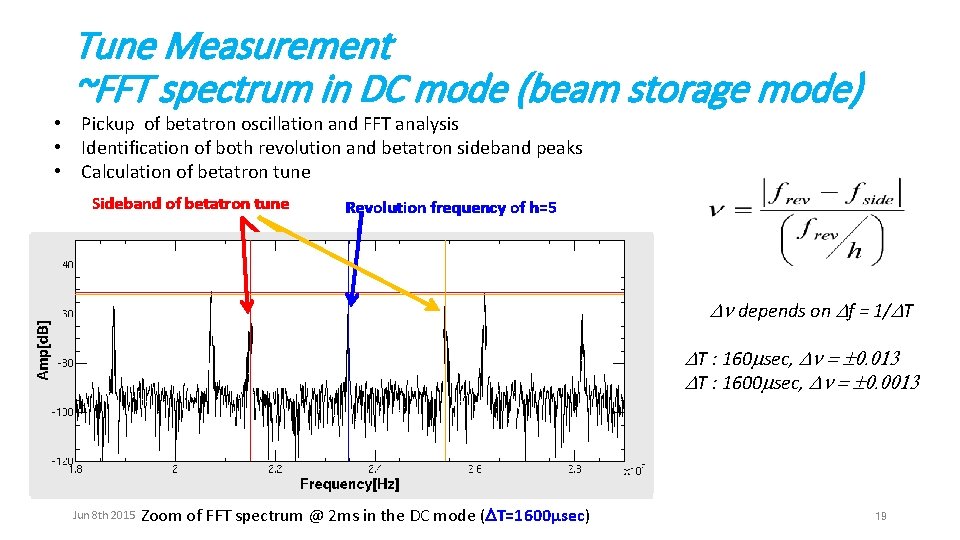
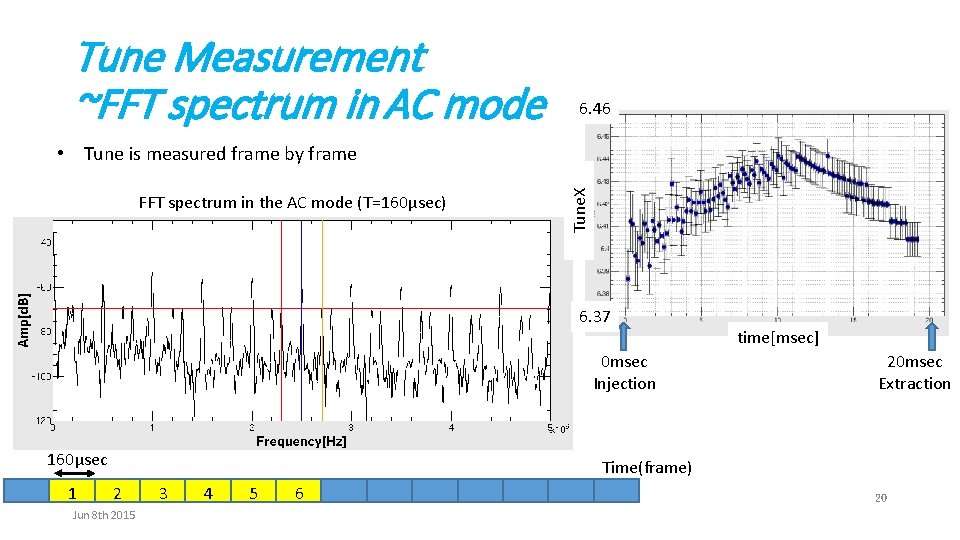
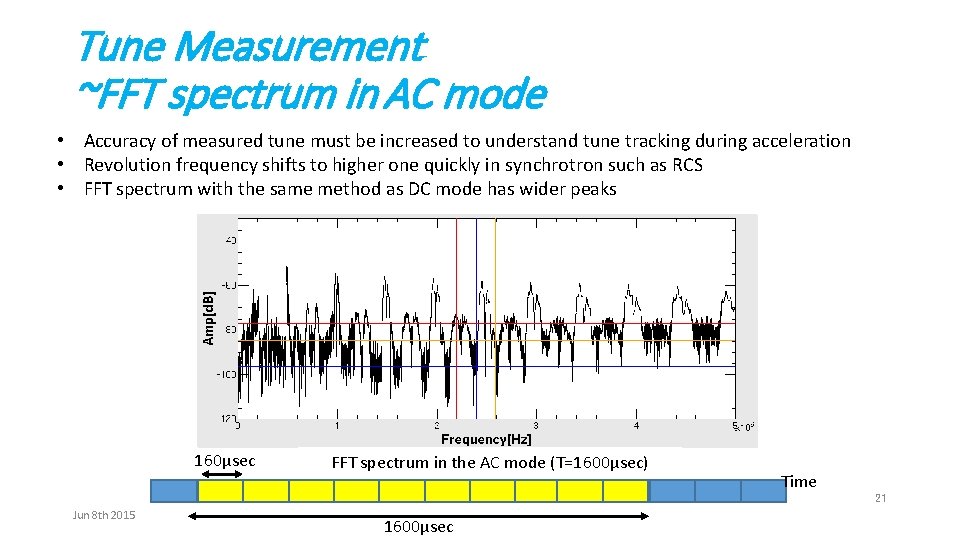
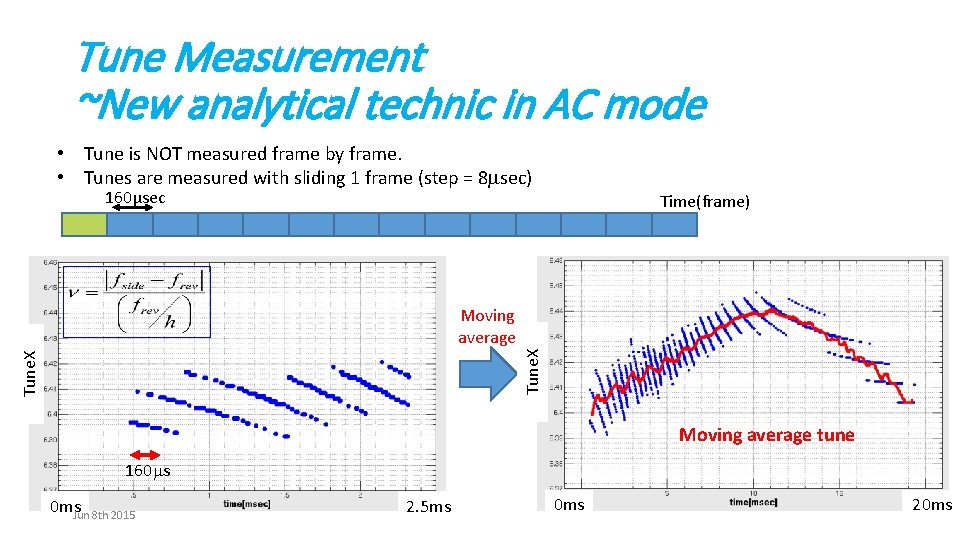
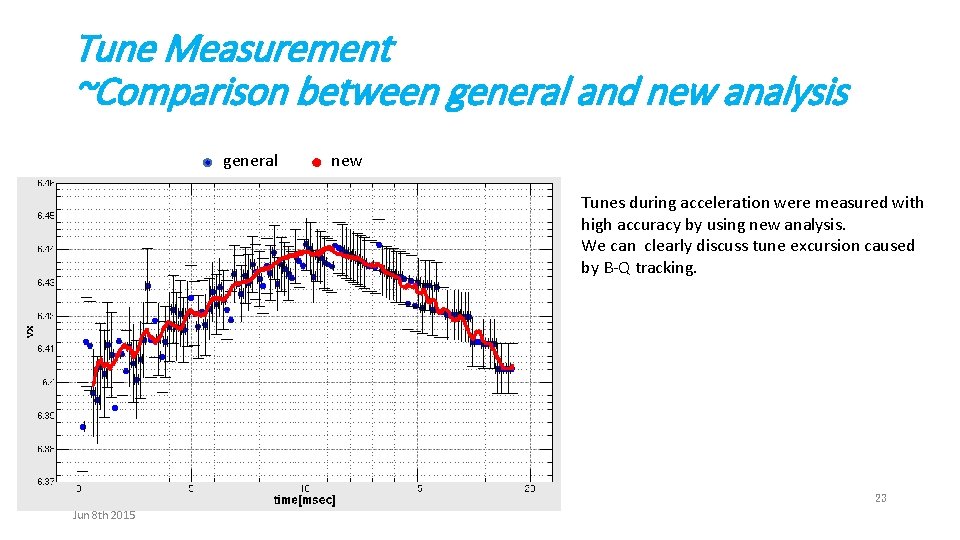
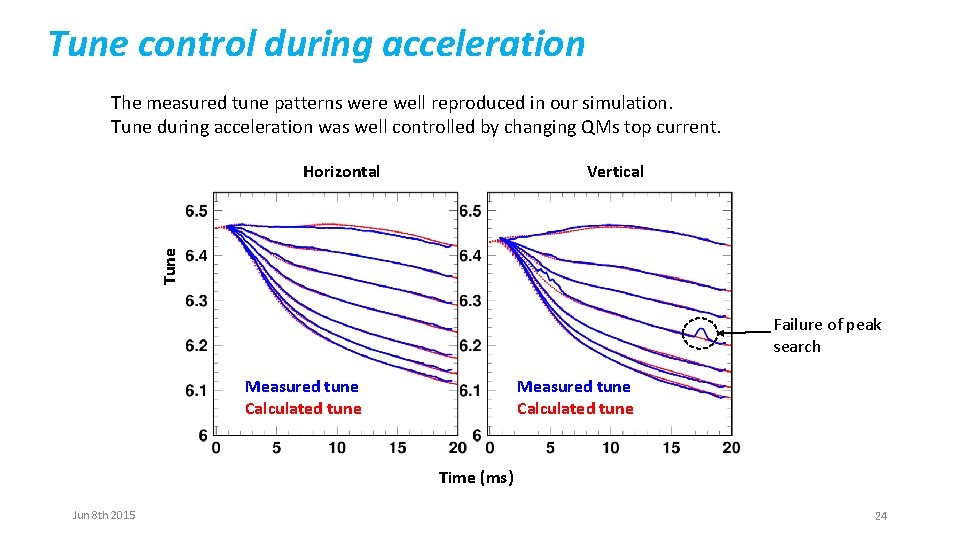
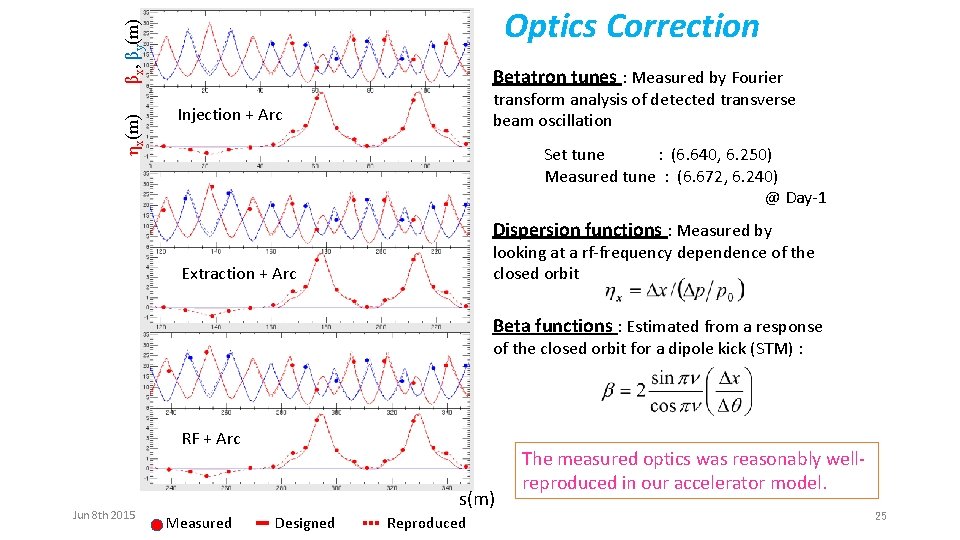
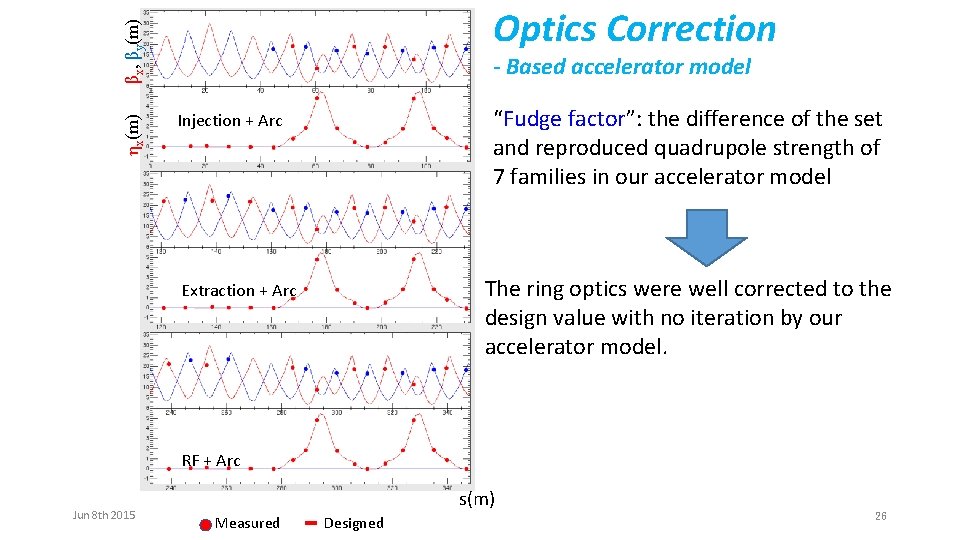
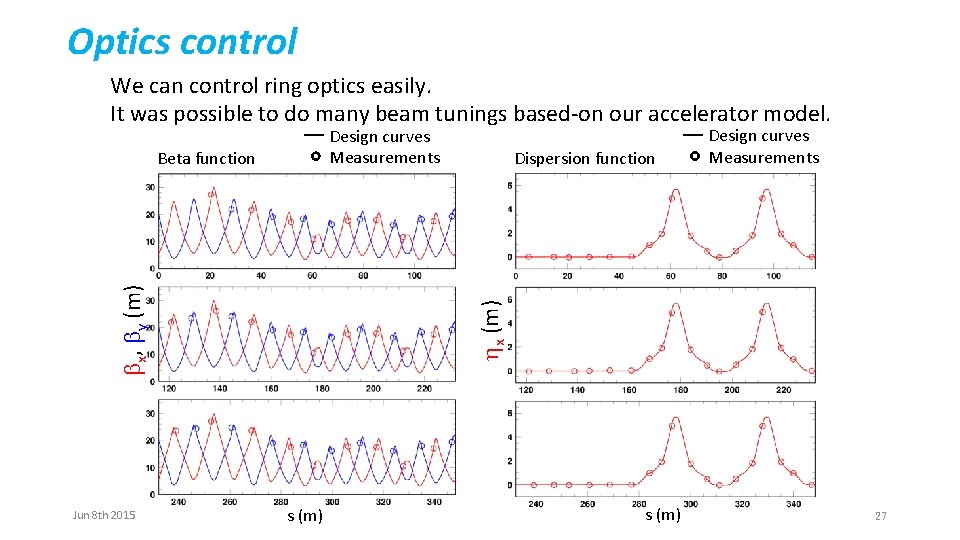
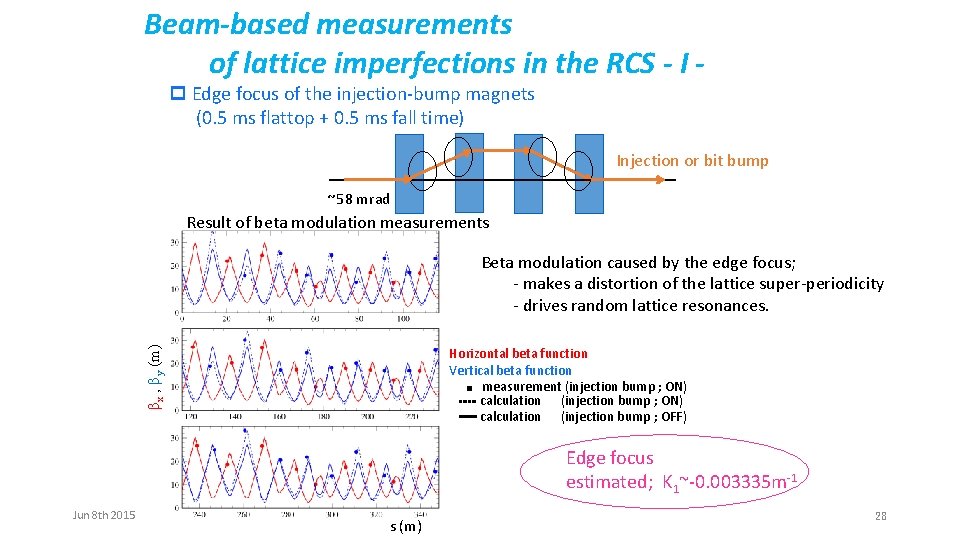
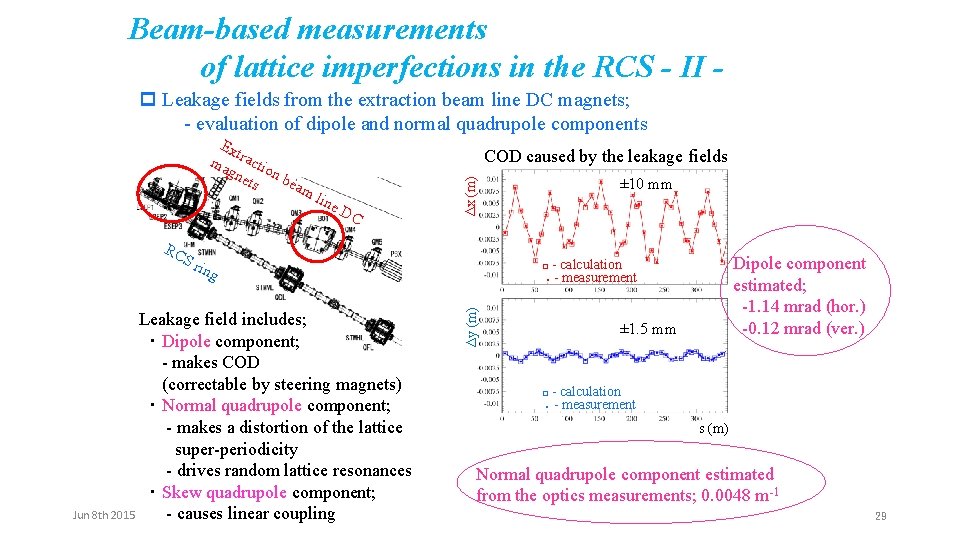
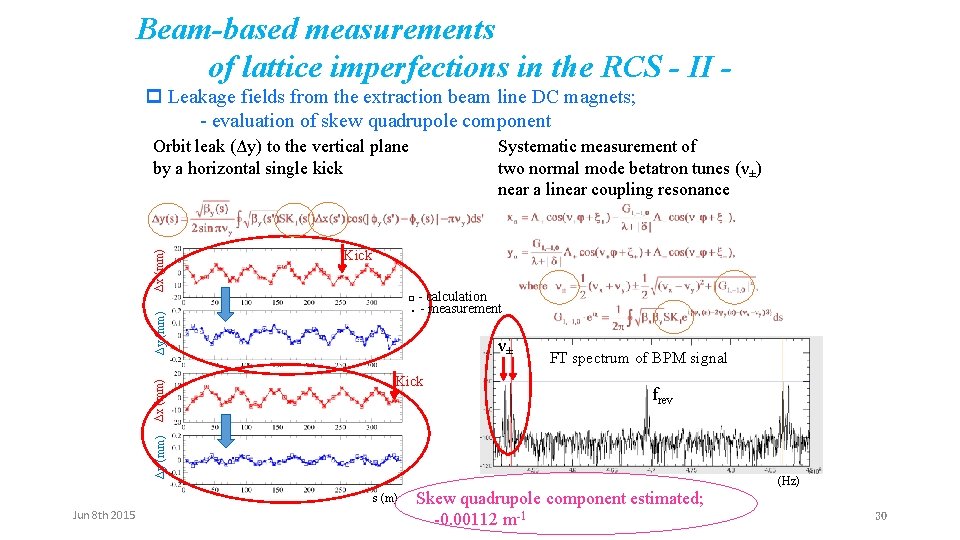
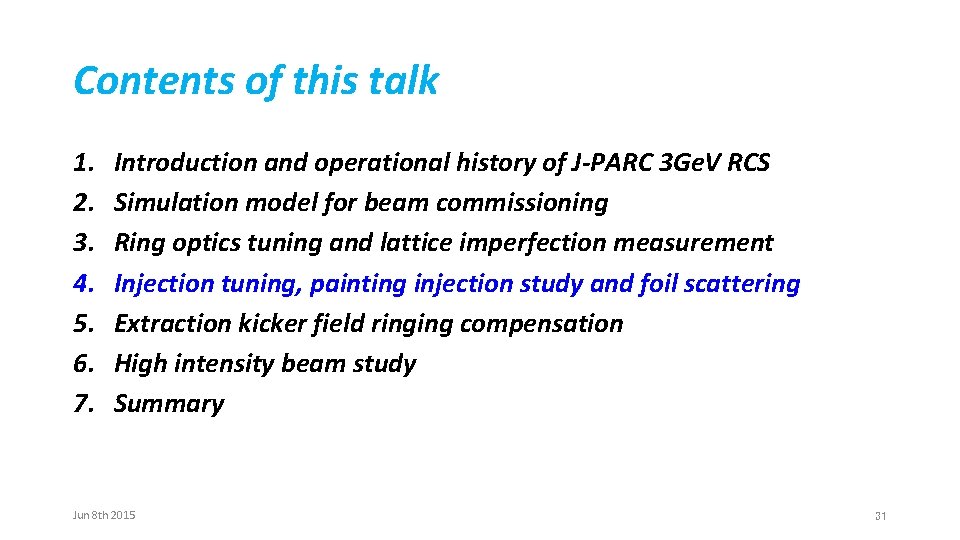
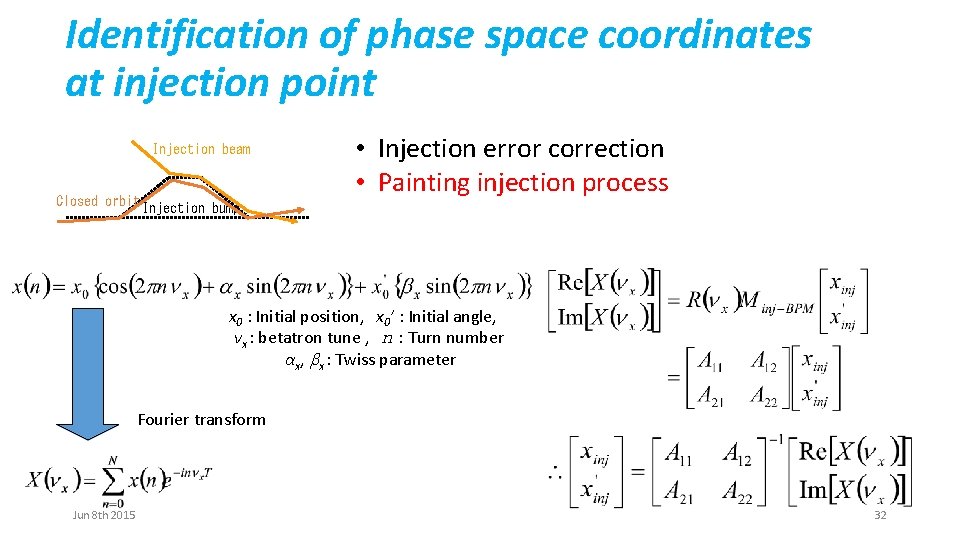
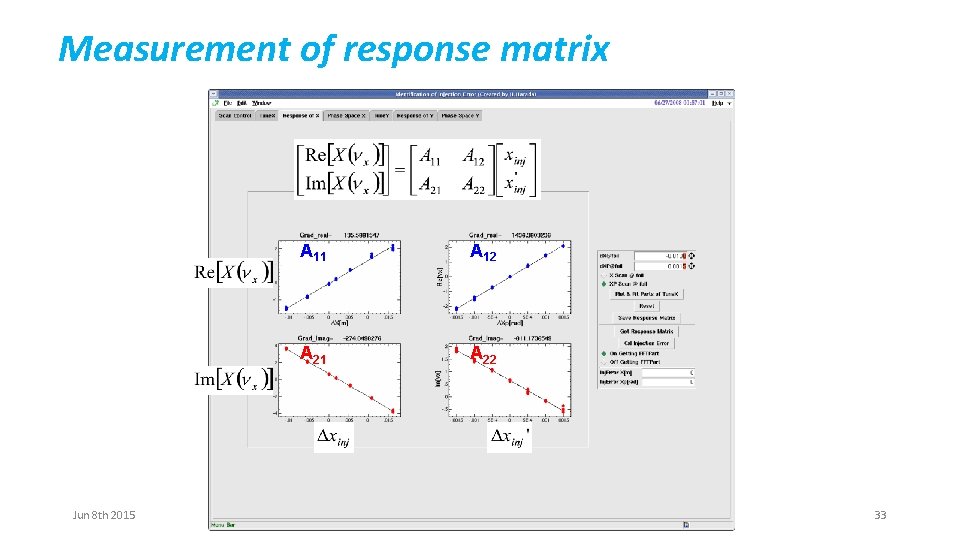
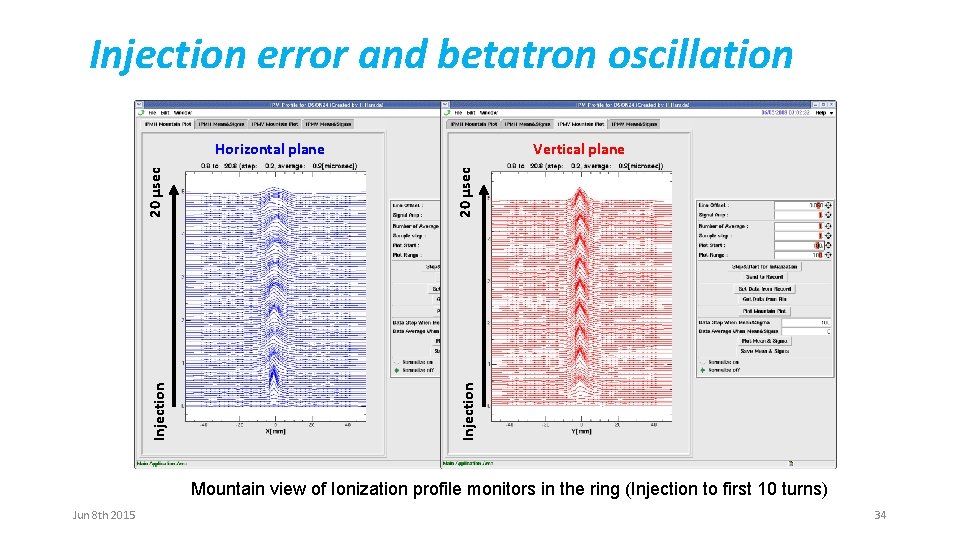
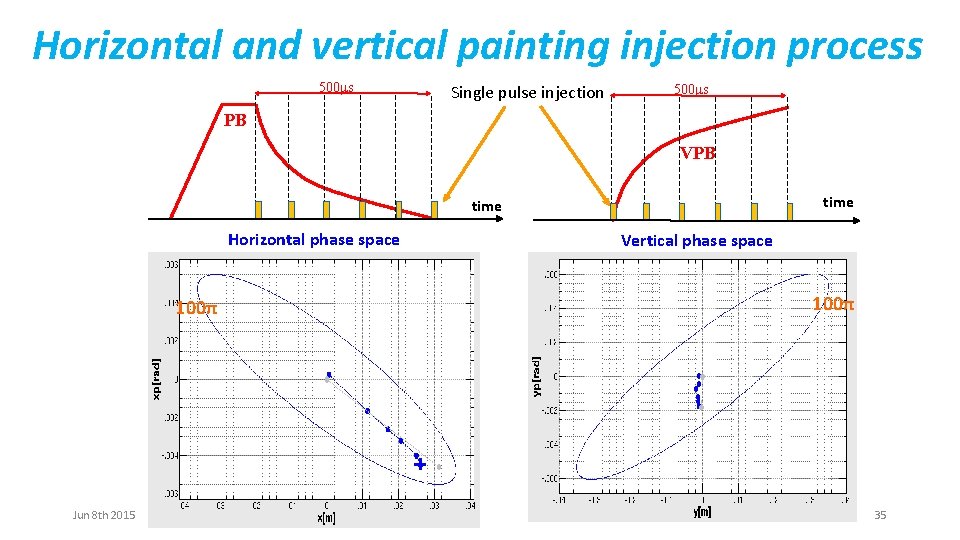
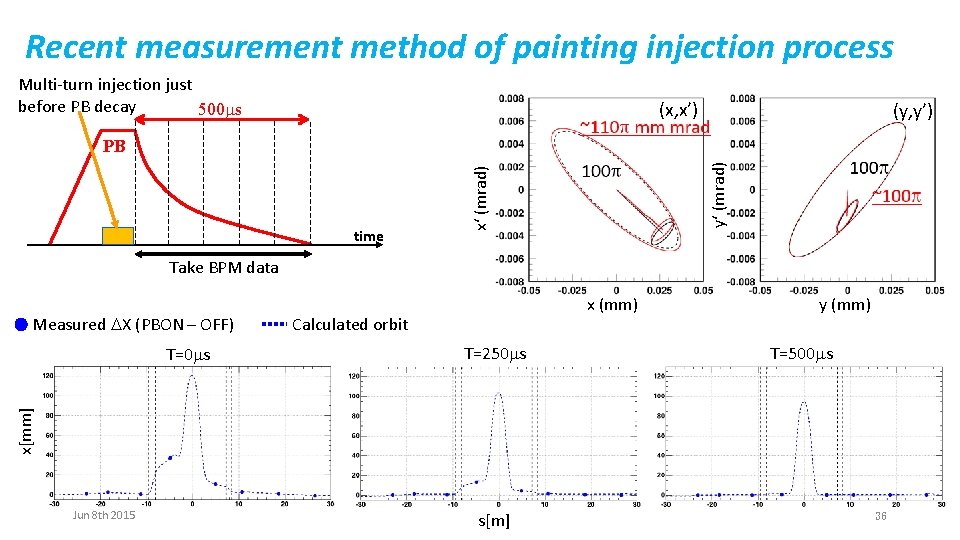
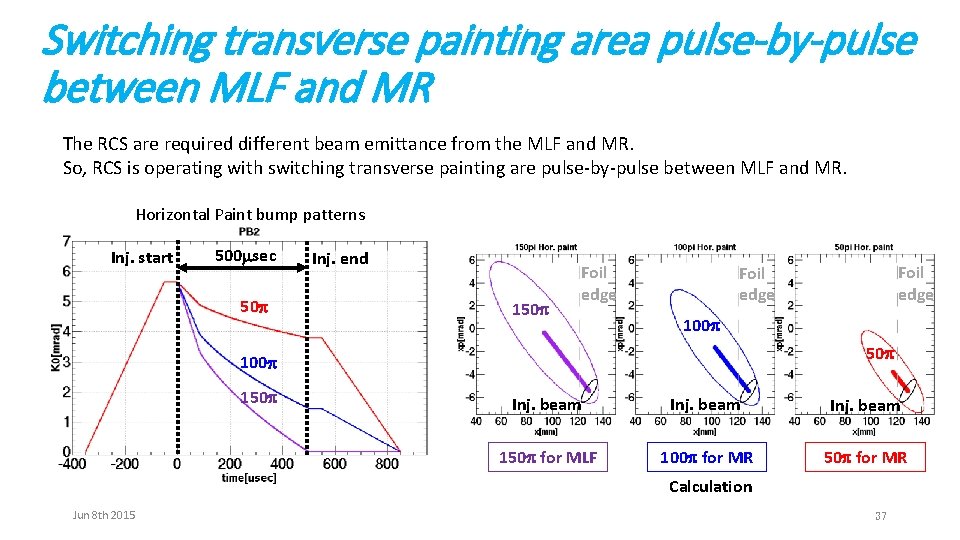
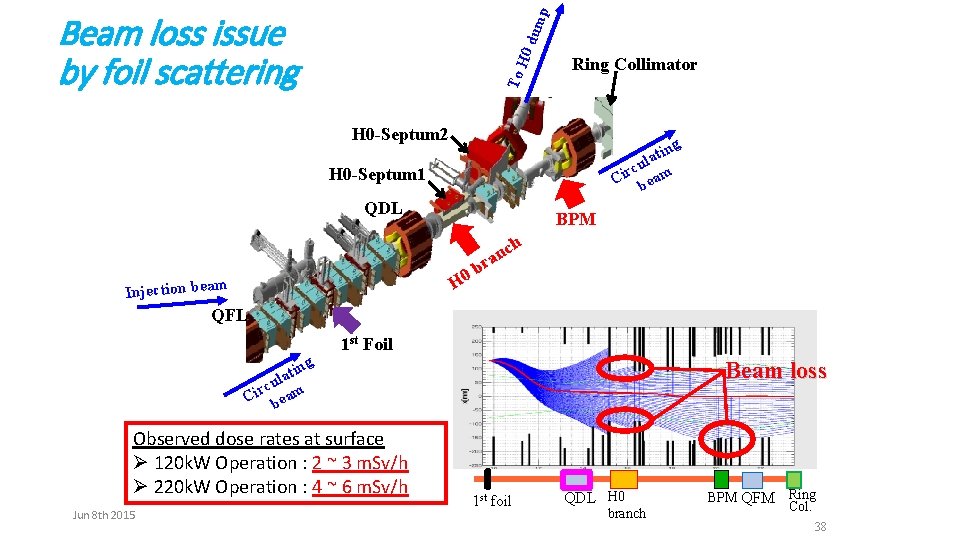
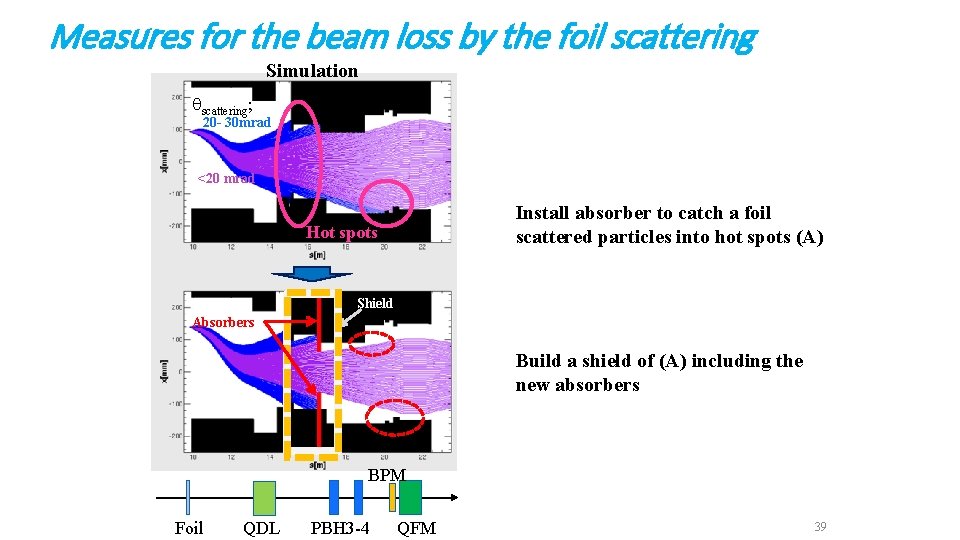
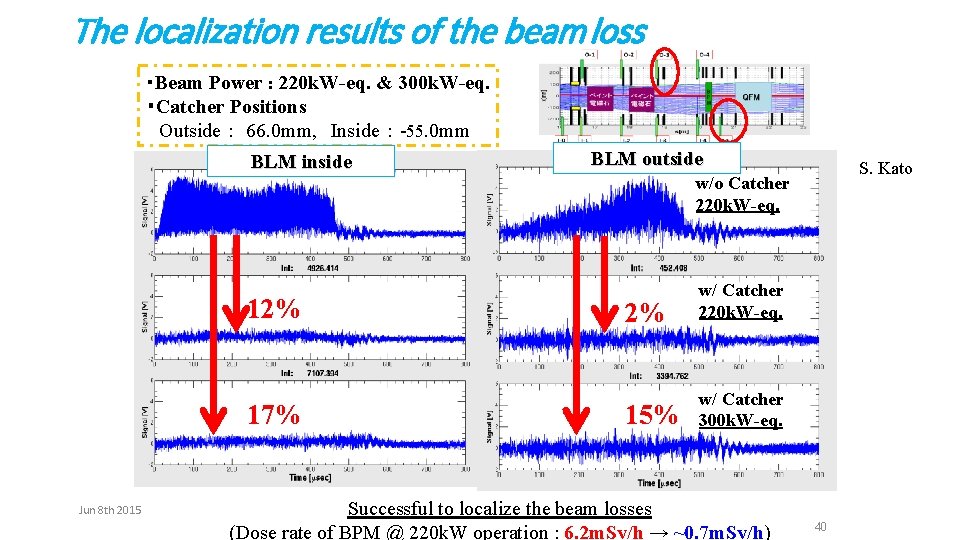
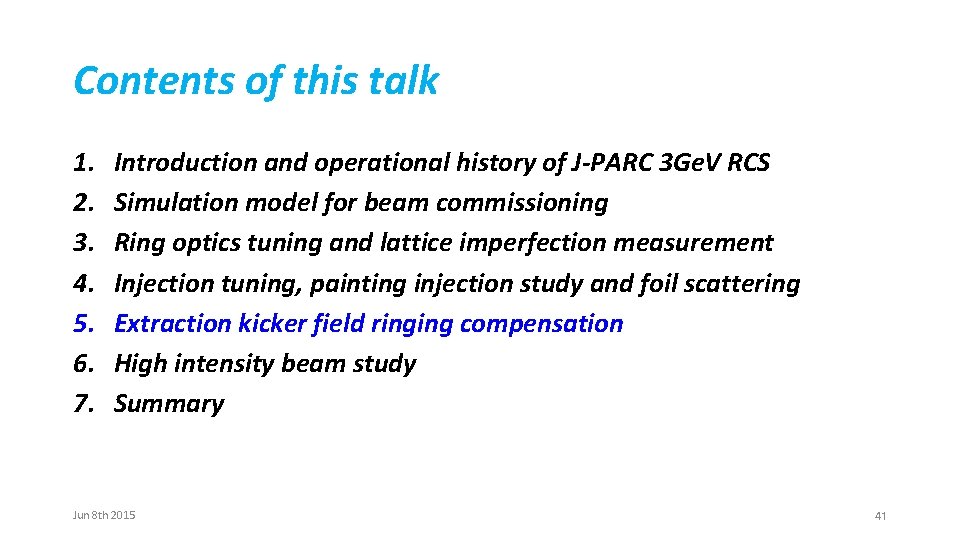
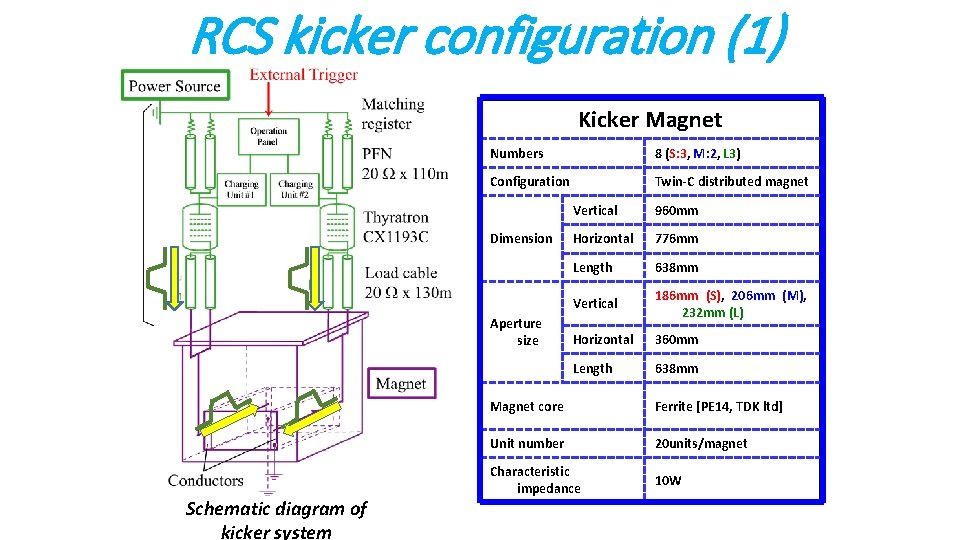
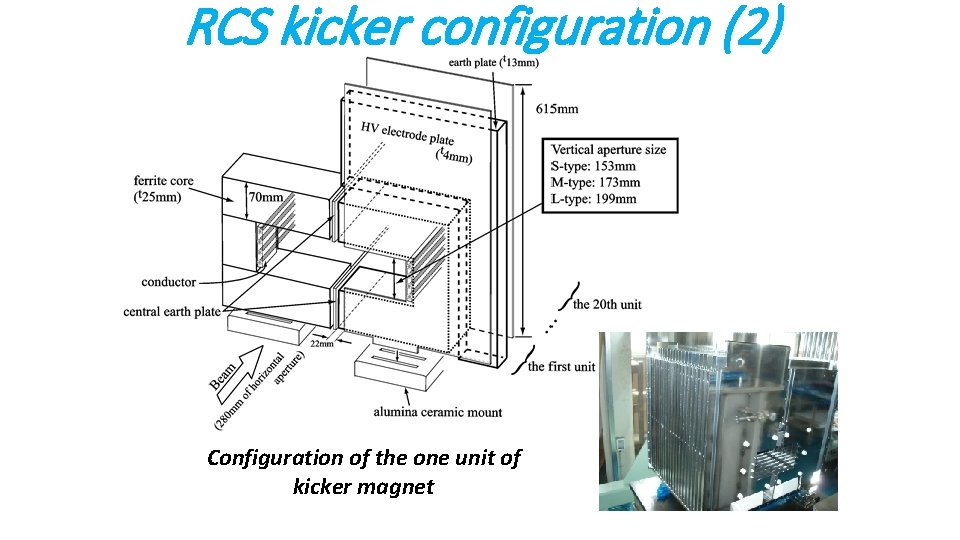
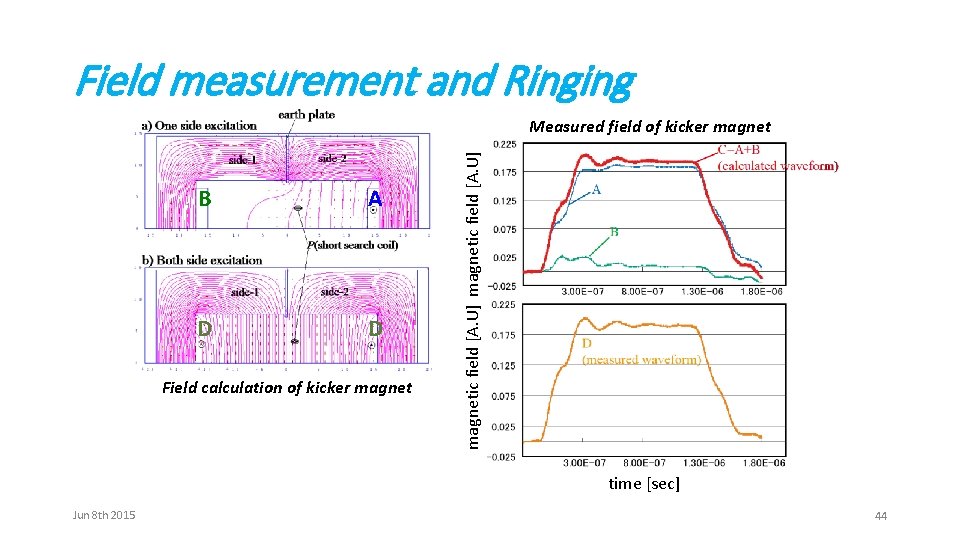
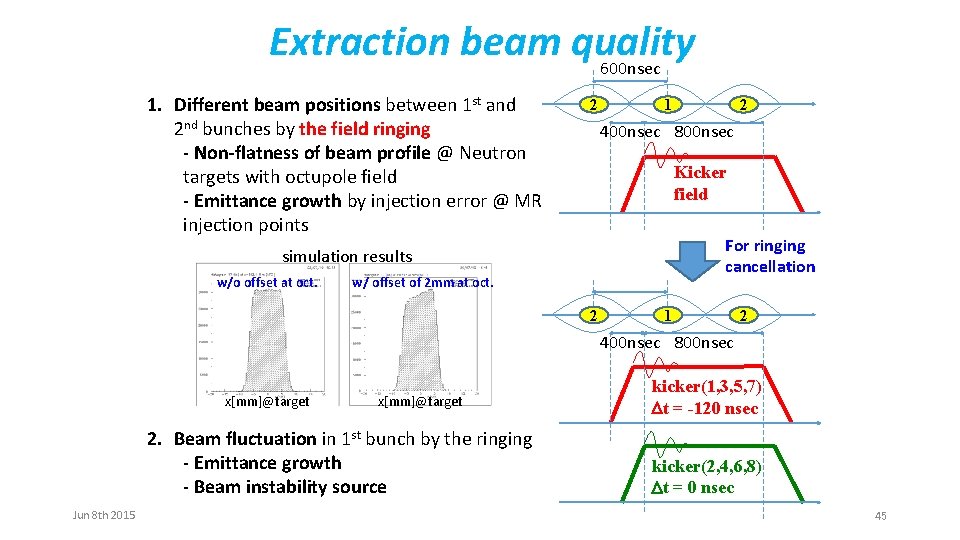
![Beam displacement measurement caused by kicker field ringing Measured Dx[mm] Beam condition : shorter Beam displacement measurement caused by kicker field ringing Measured Dx[mm] Beam condition : shorter](https://slidetodoc.com/presentation_image_h2/5d0aad23d25bb3e3627177fa406188e3/image-46.jpg)
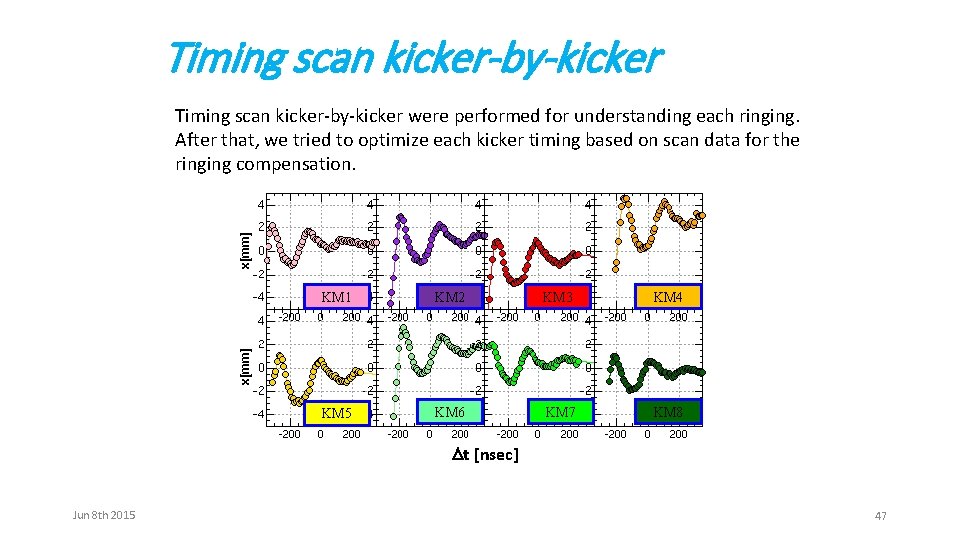
![ID ① ΔT ② ΔT ① KM 1 0 nsec 10 nsec Measured Dx[mm] ID ① ΔT ② ΔT ① KM 1 0 nsec 10 nsec Measured Dx[mm]](https://slidetodoc.com/presentation_image_h2/5d0aad23d25bb3e3627177fa406188e3/image-48.jpg)
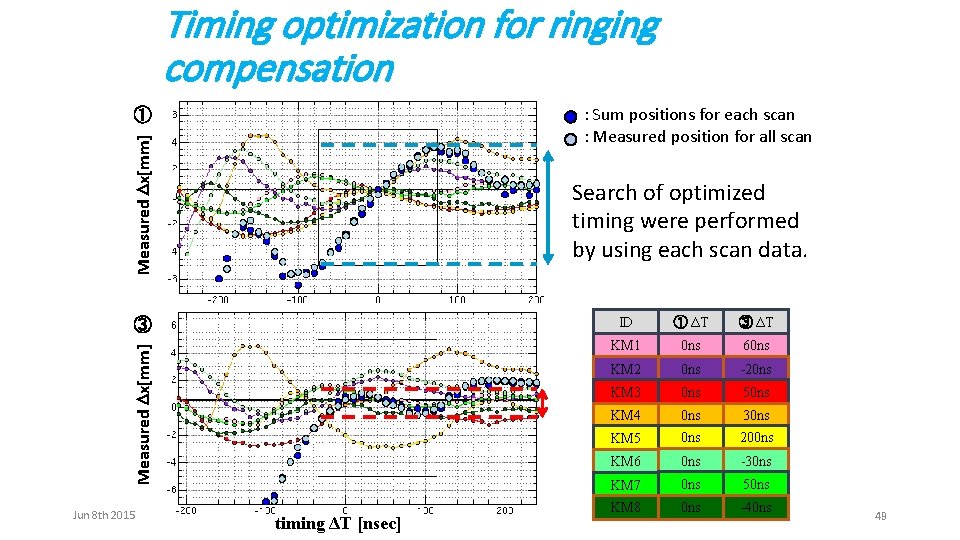
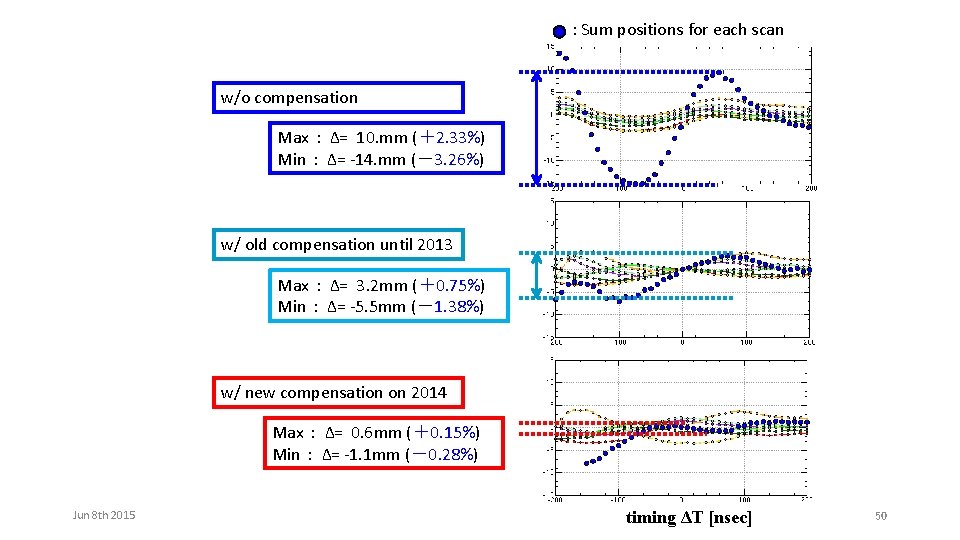
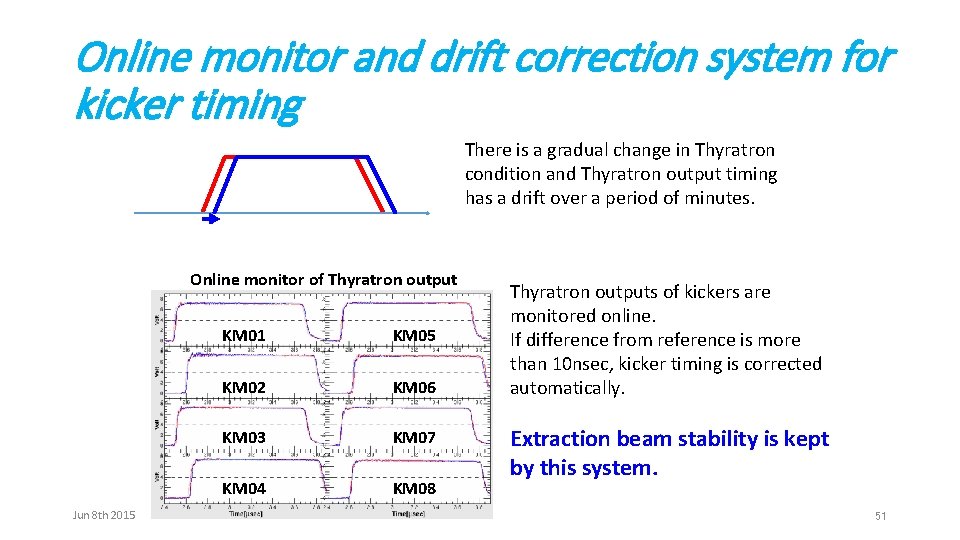
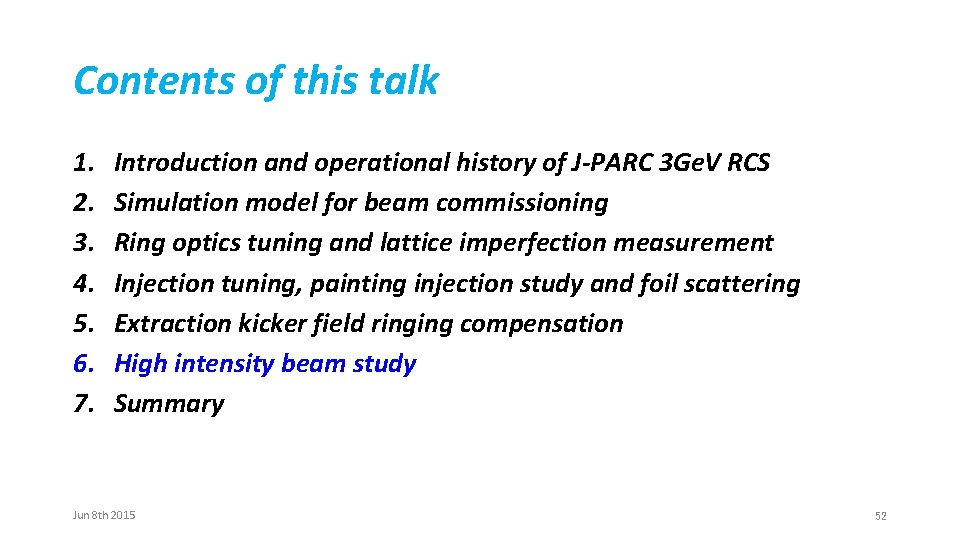
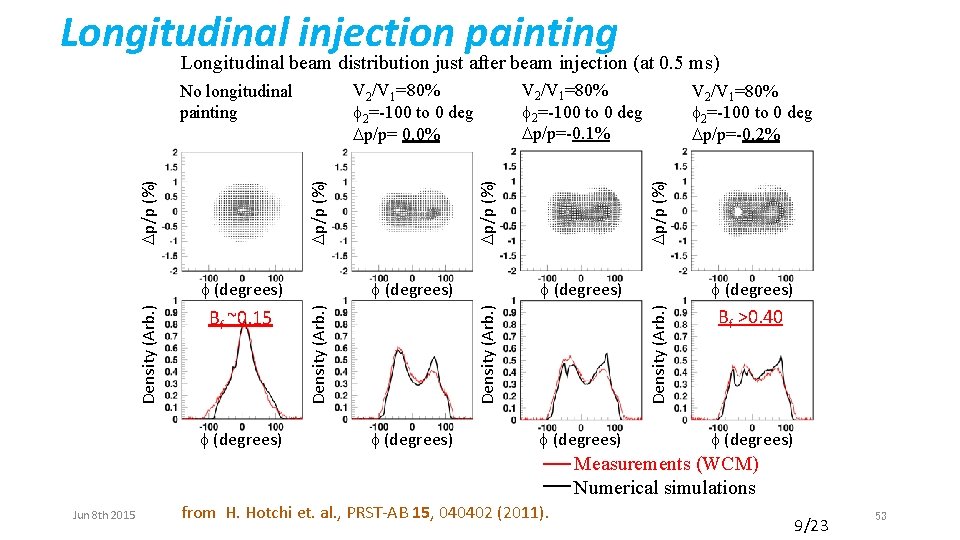
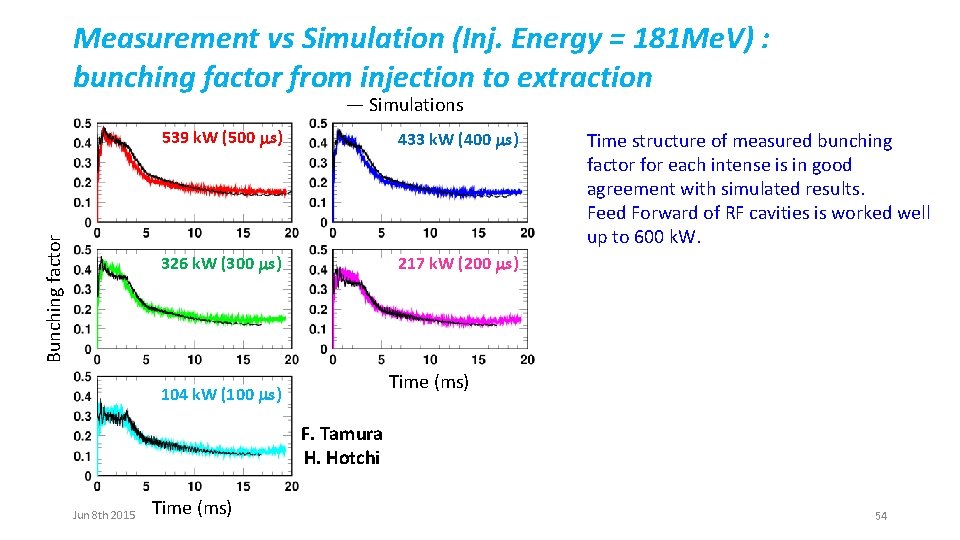
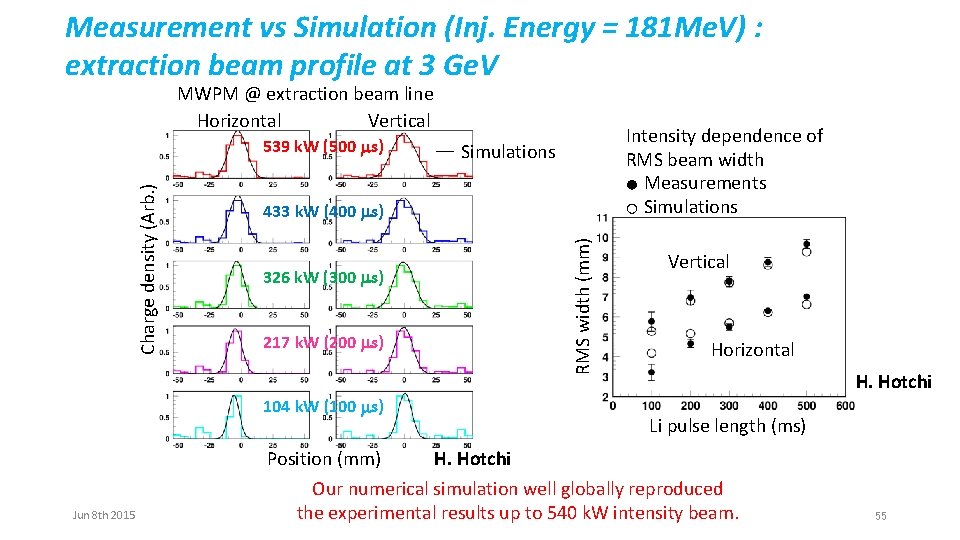
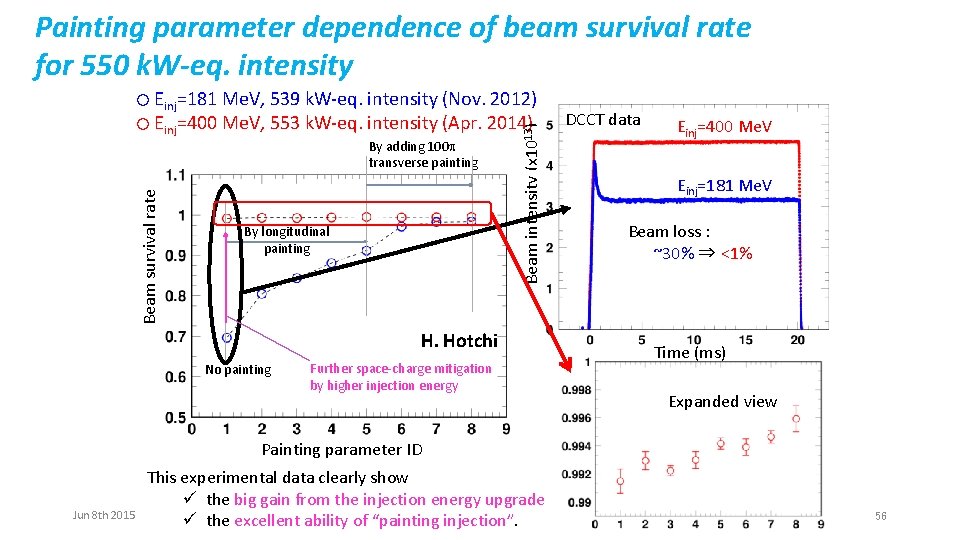
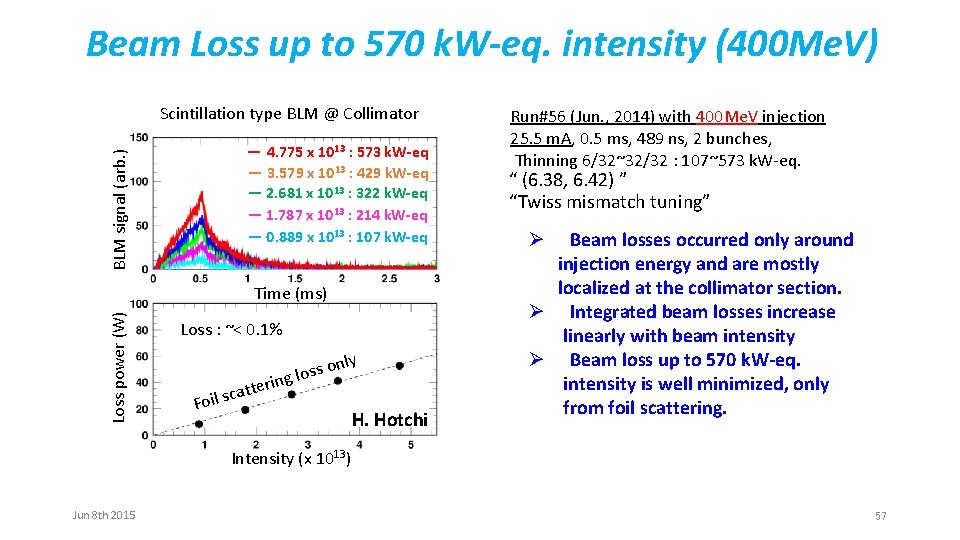
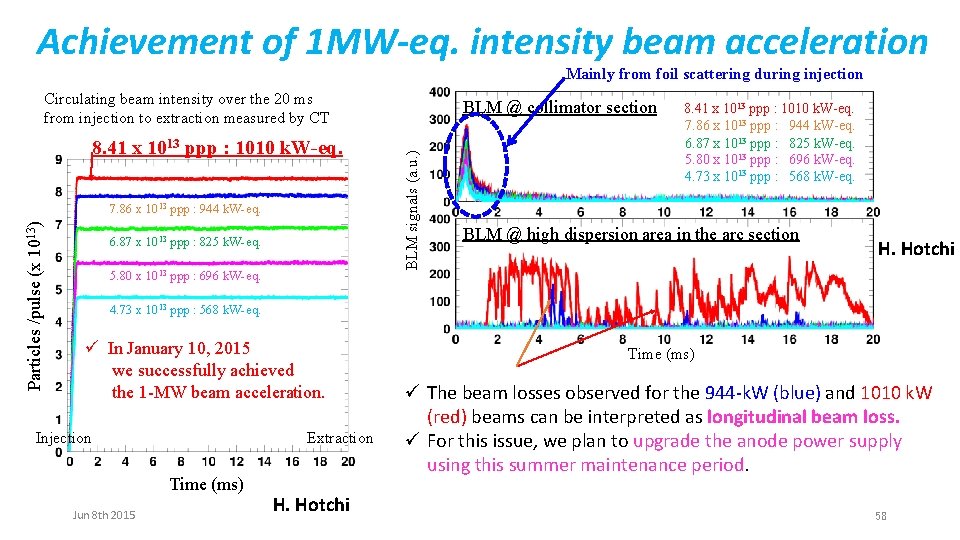
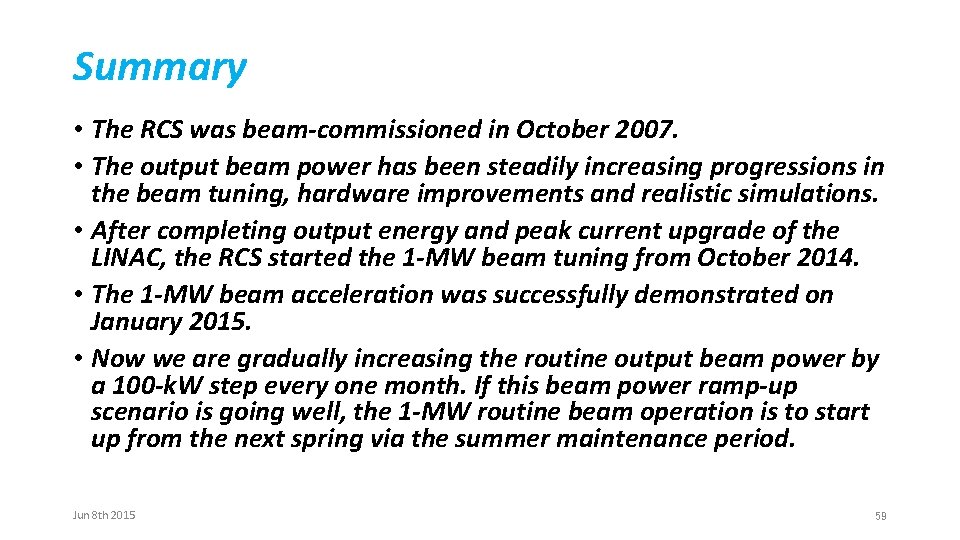
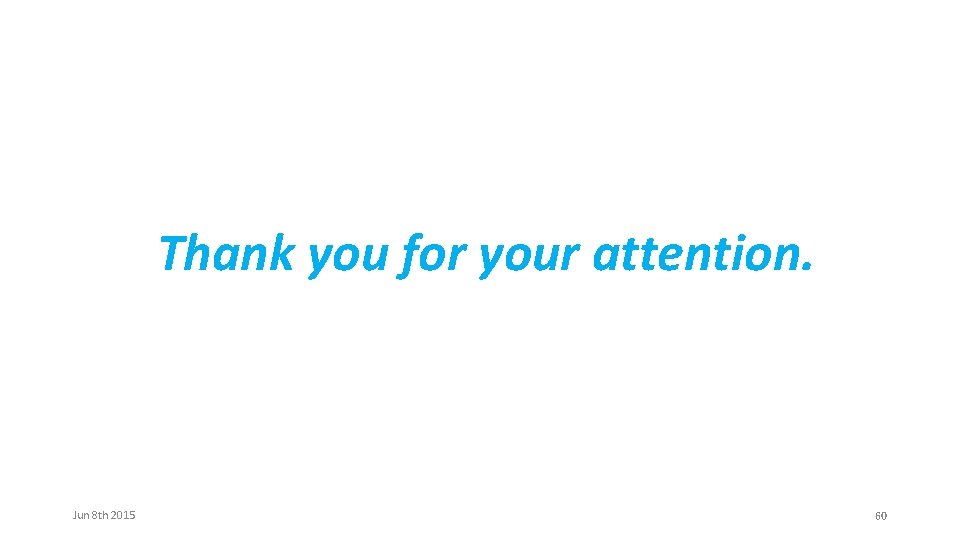
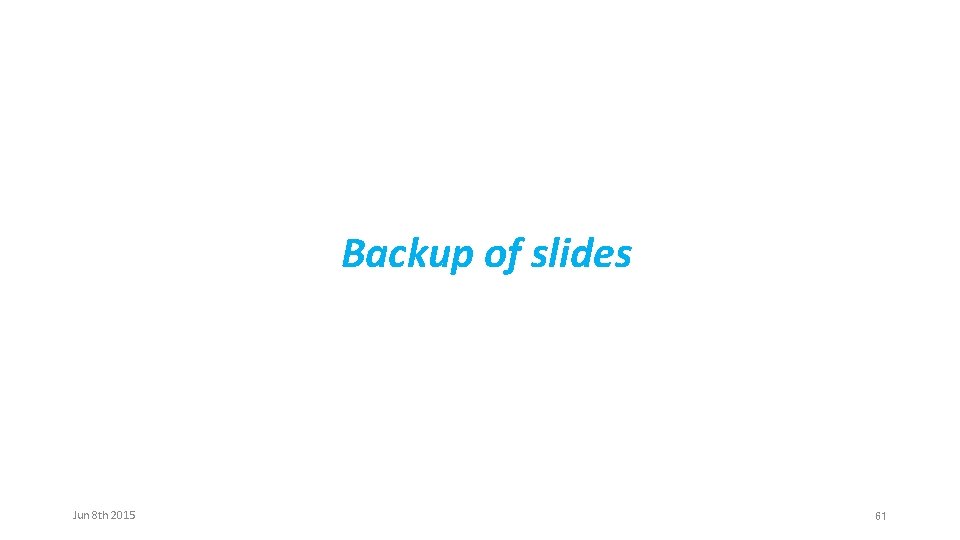
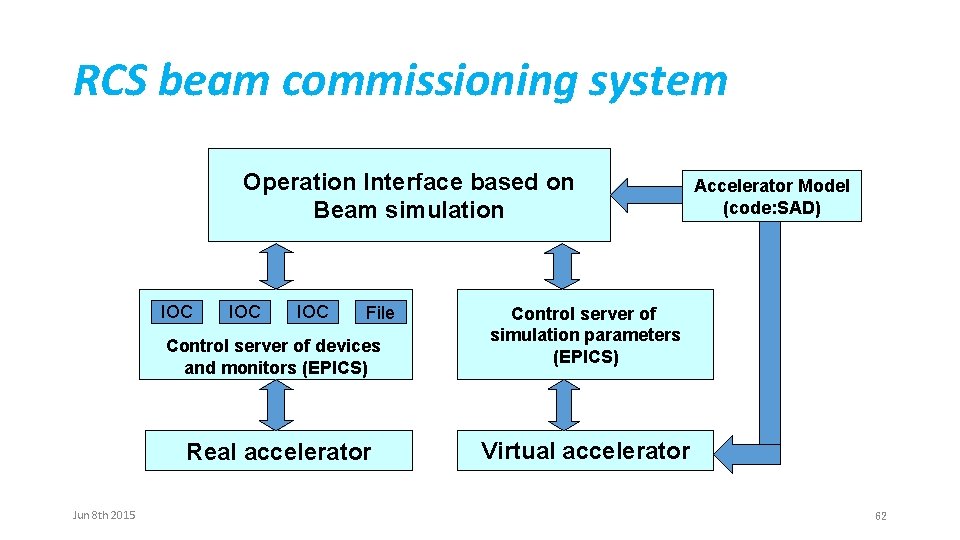
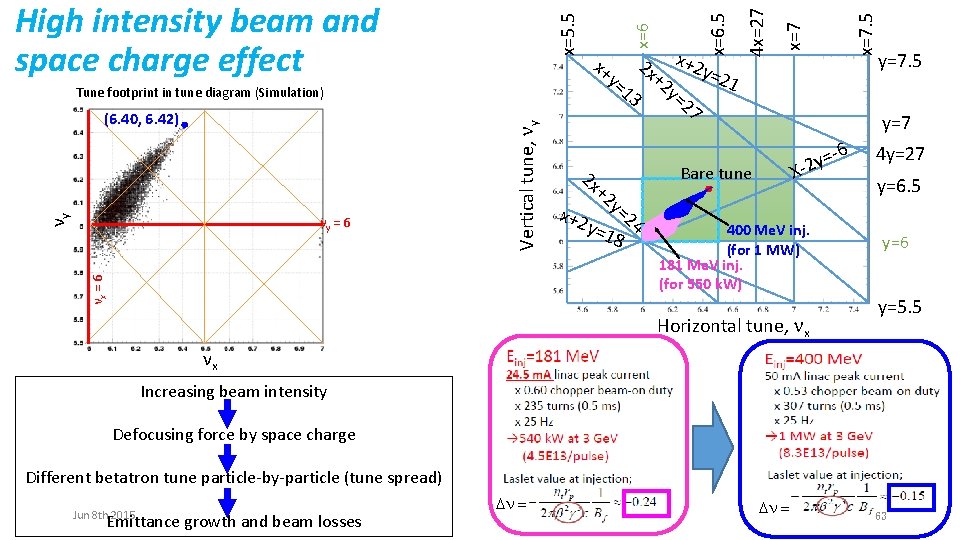
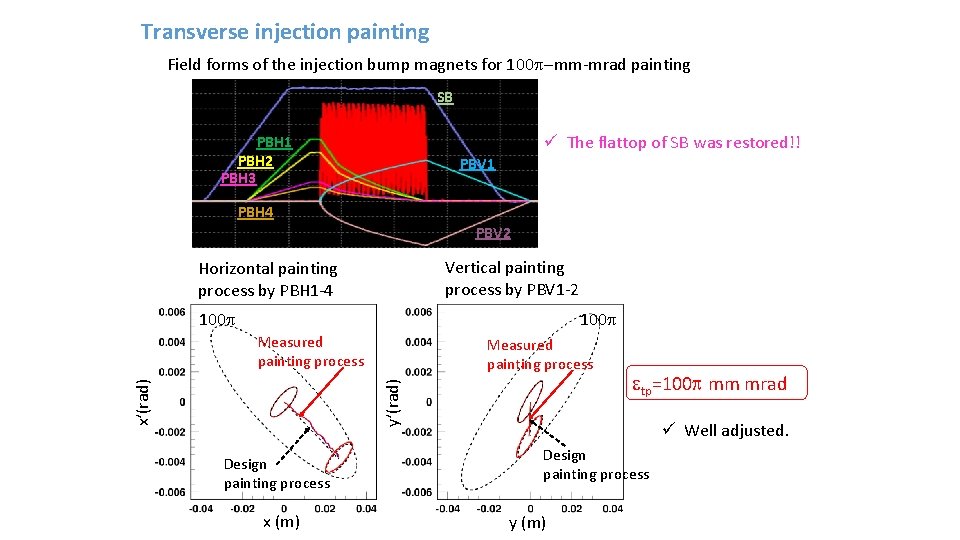
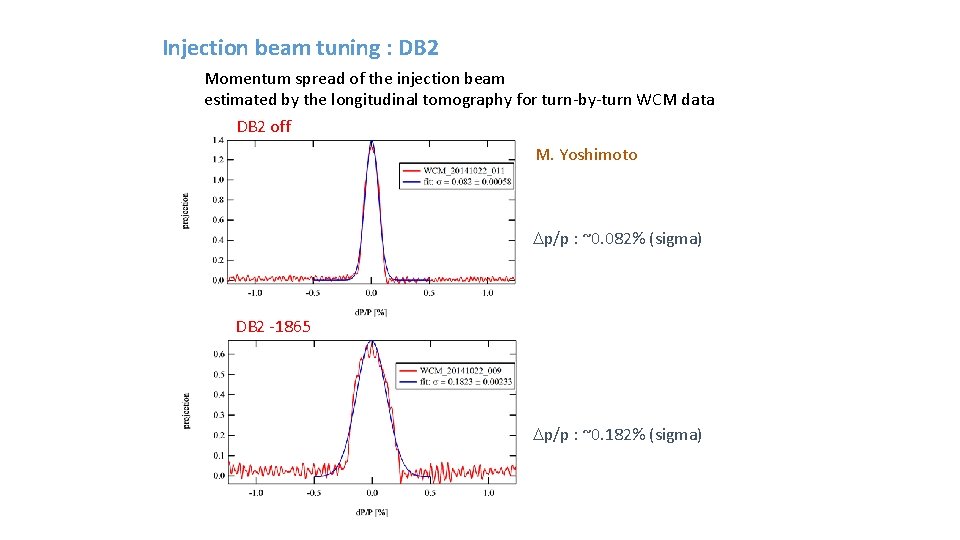
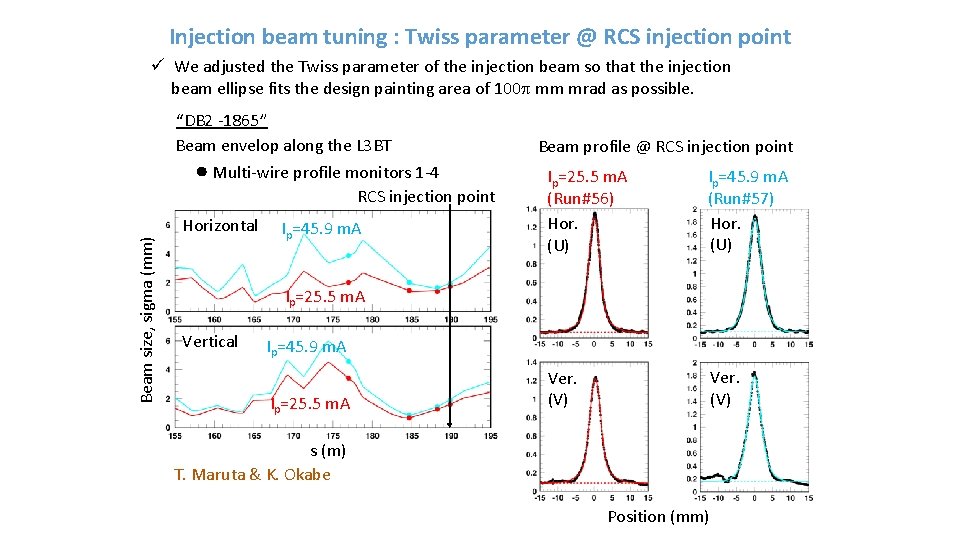
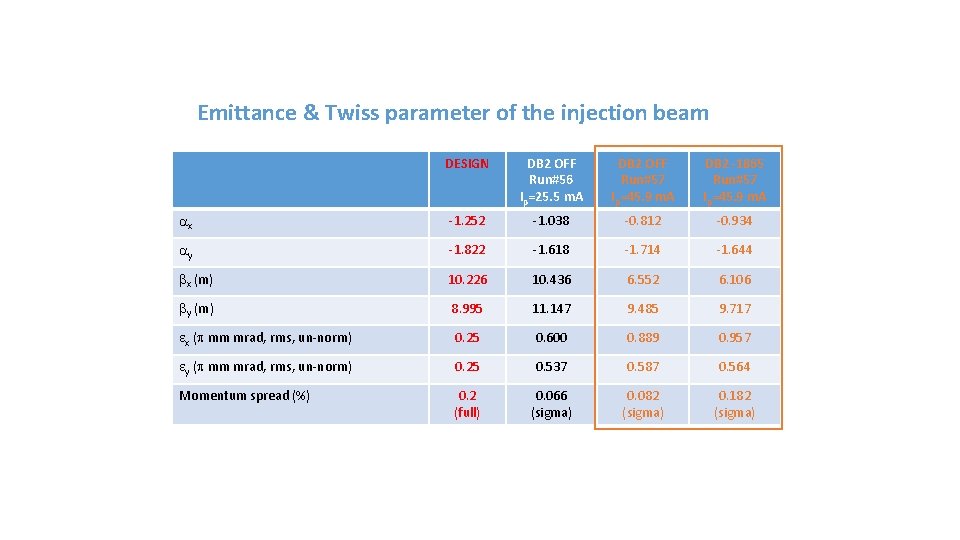
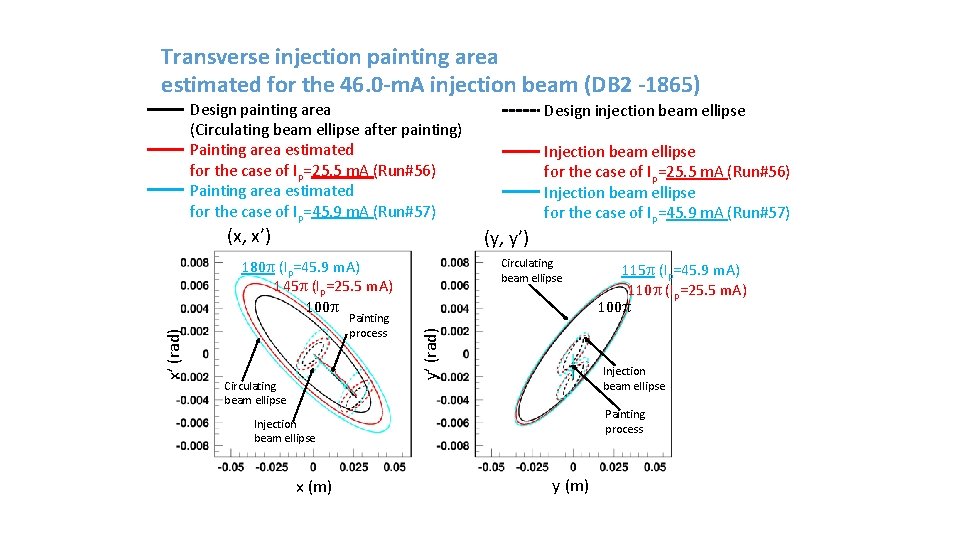
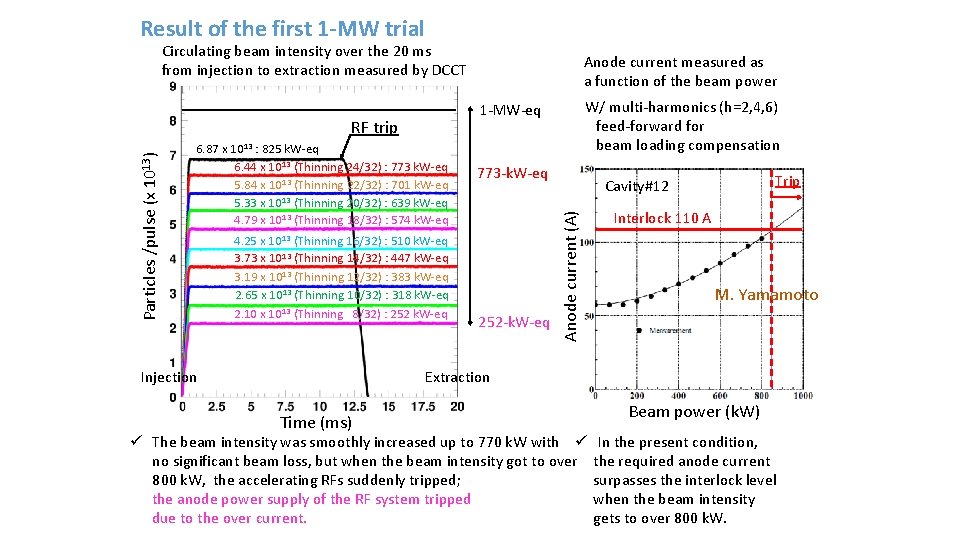
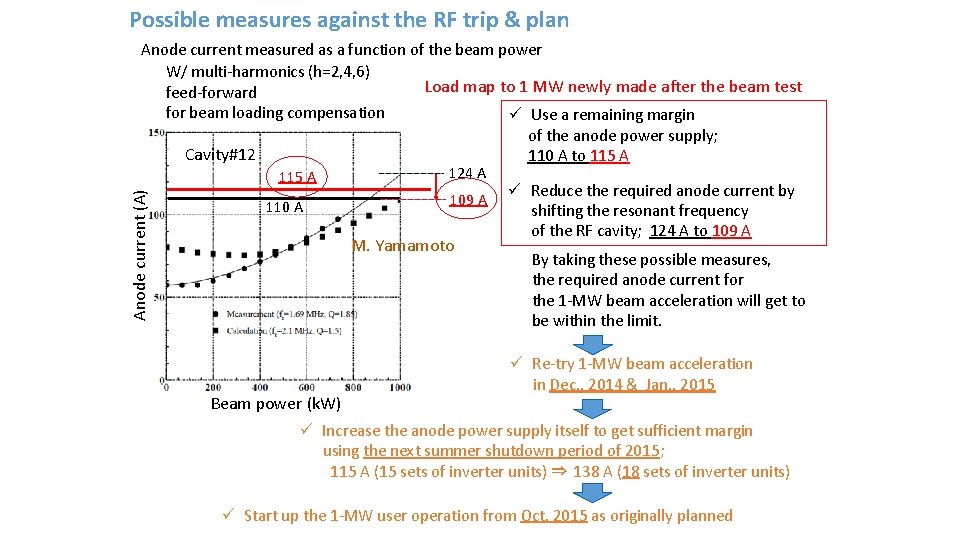
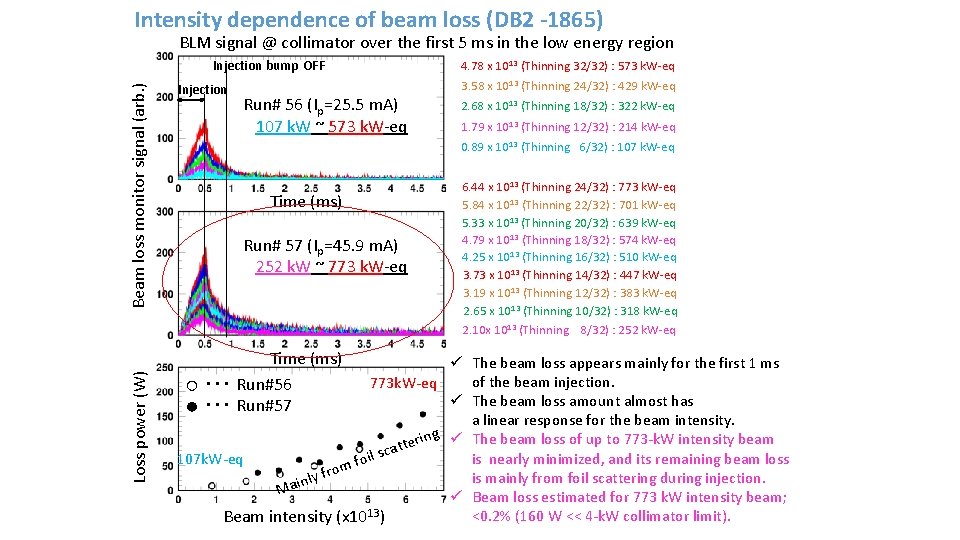
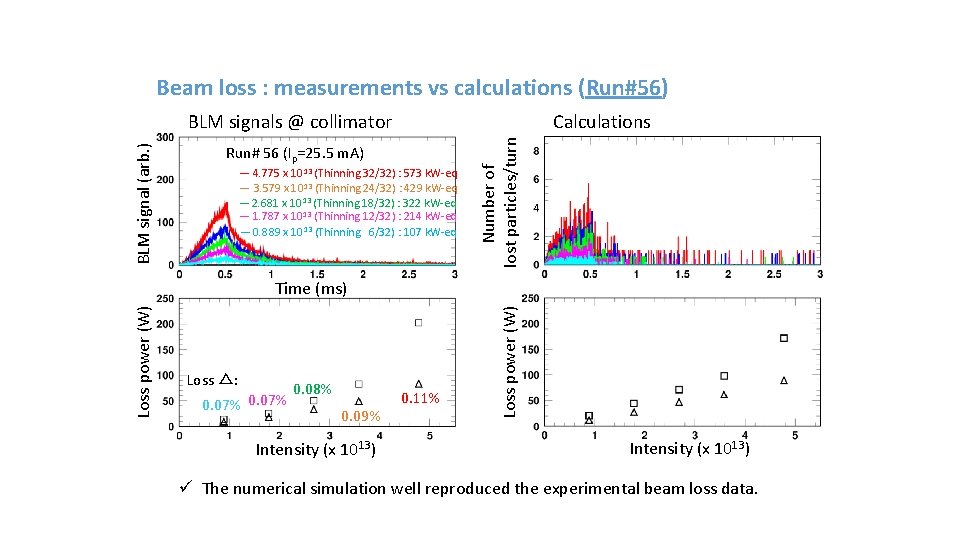
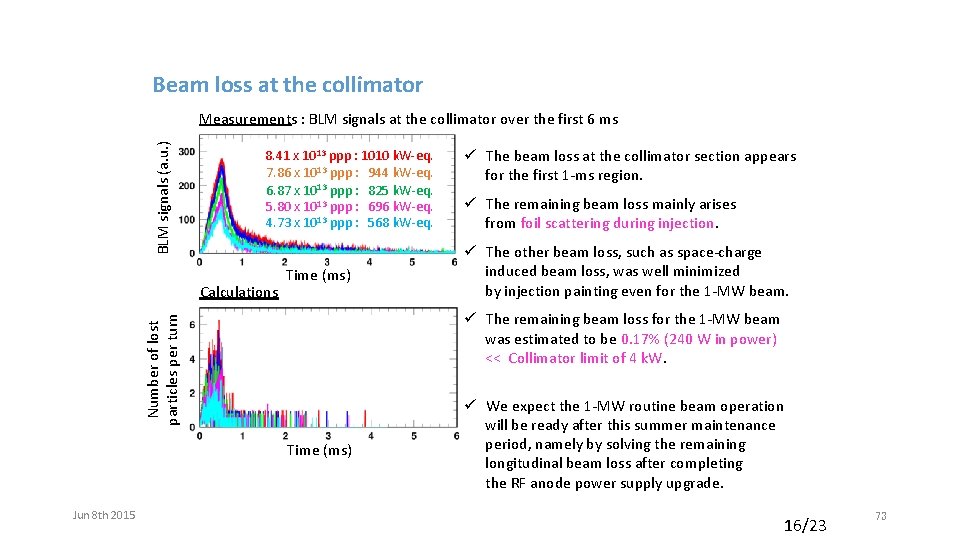
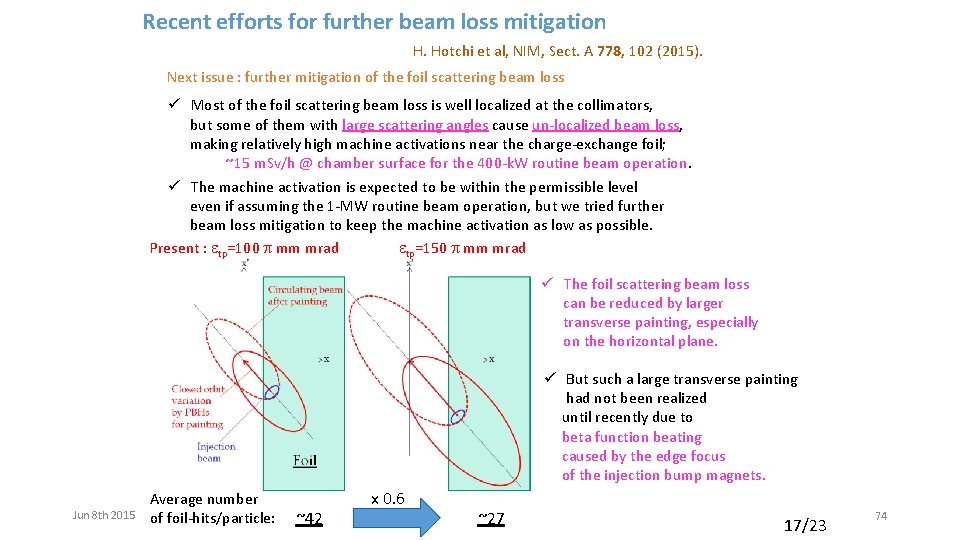
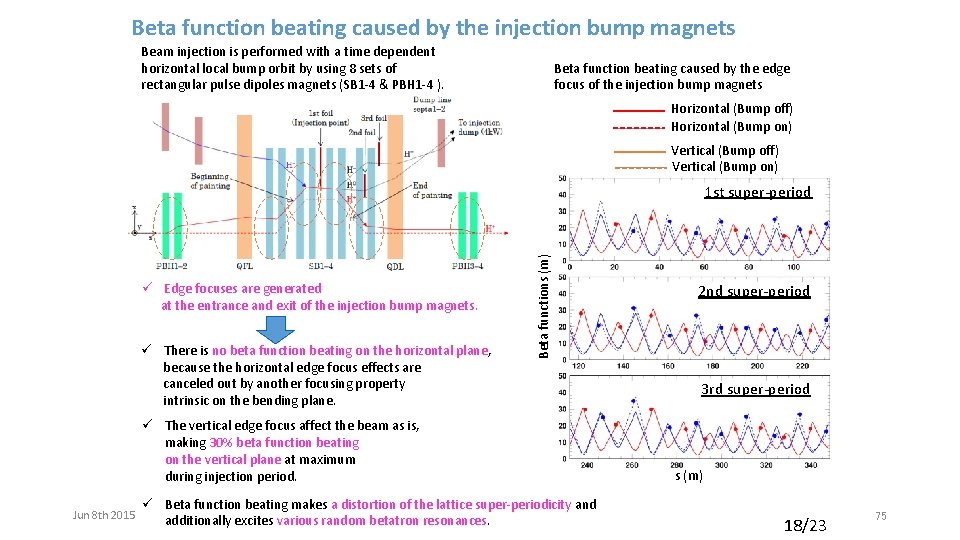
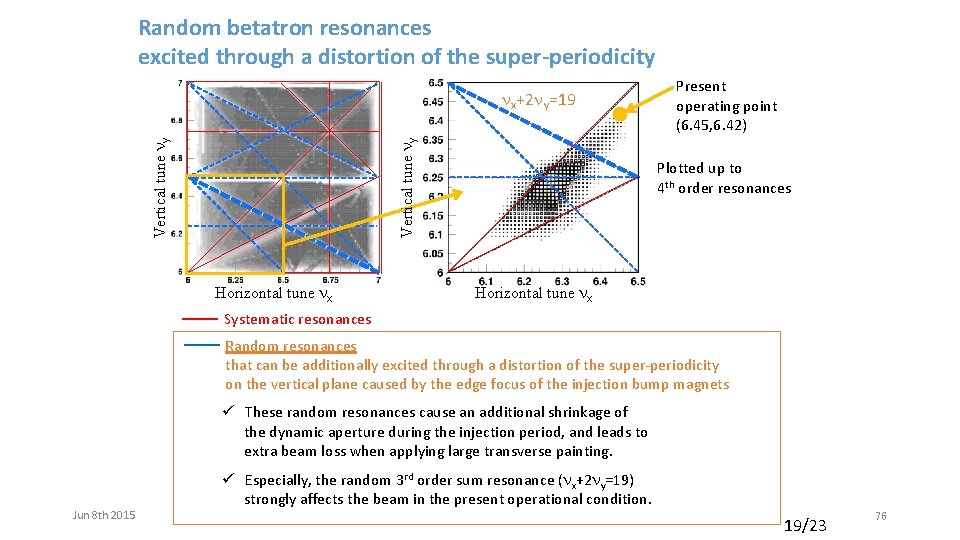
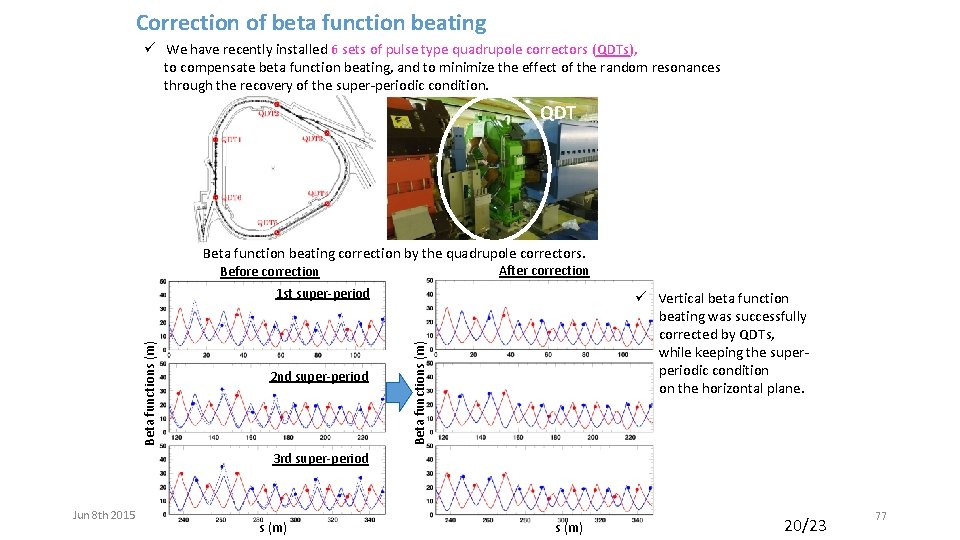
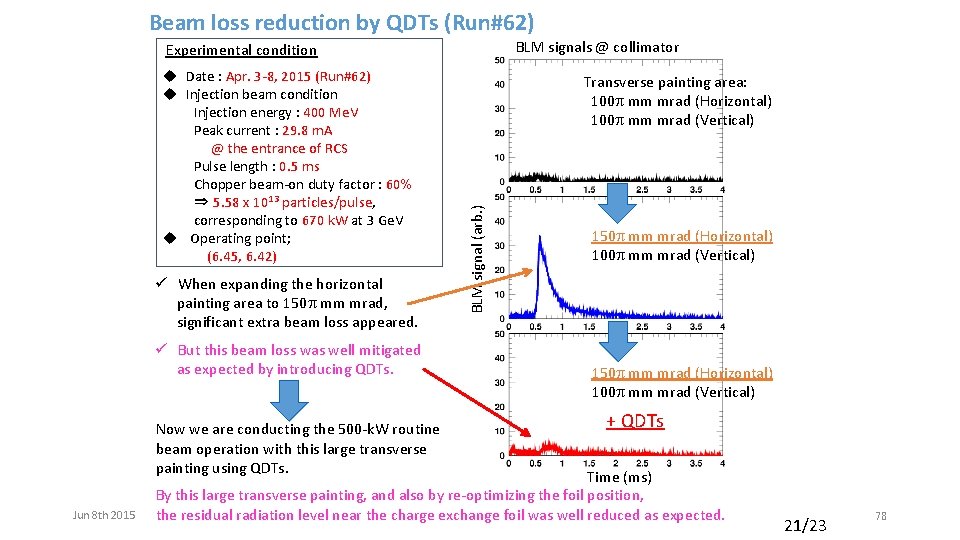
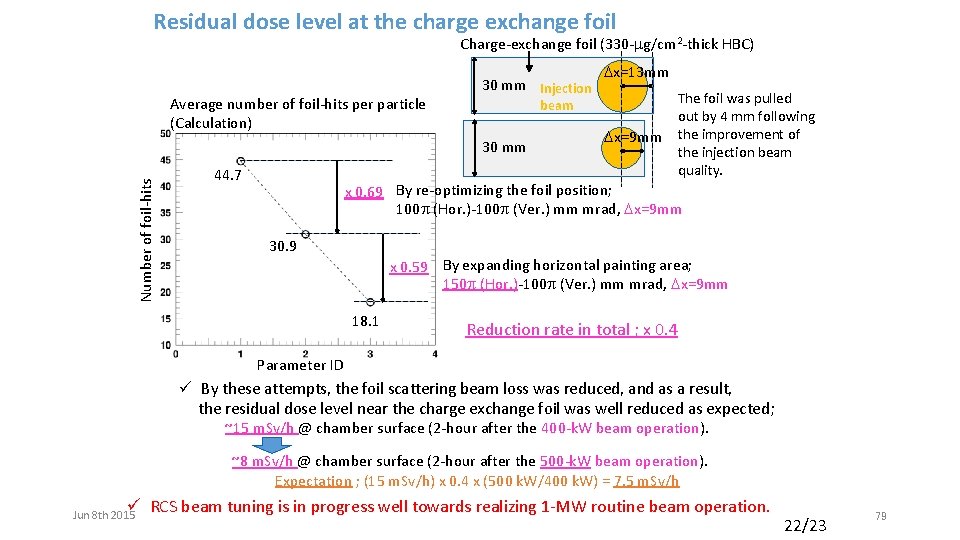
- Slides: 79
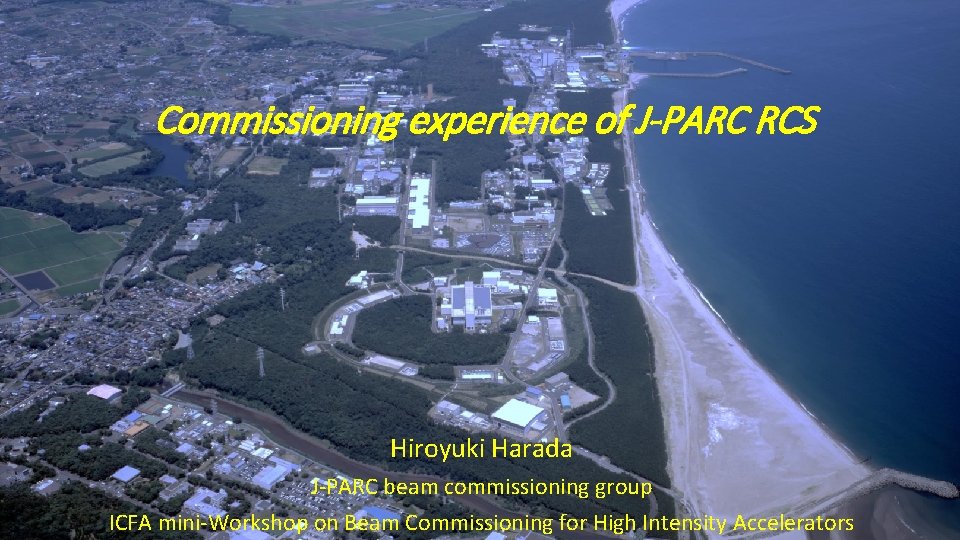
Commissioning experience of J-PARC RCS Hiroyuki Harada J-PARC beam commissioning group ICFA mini-Workshop on Beam Commissioning for High Intensity Accelerators
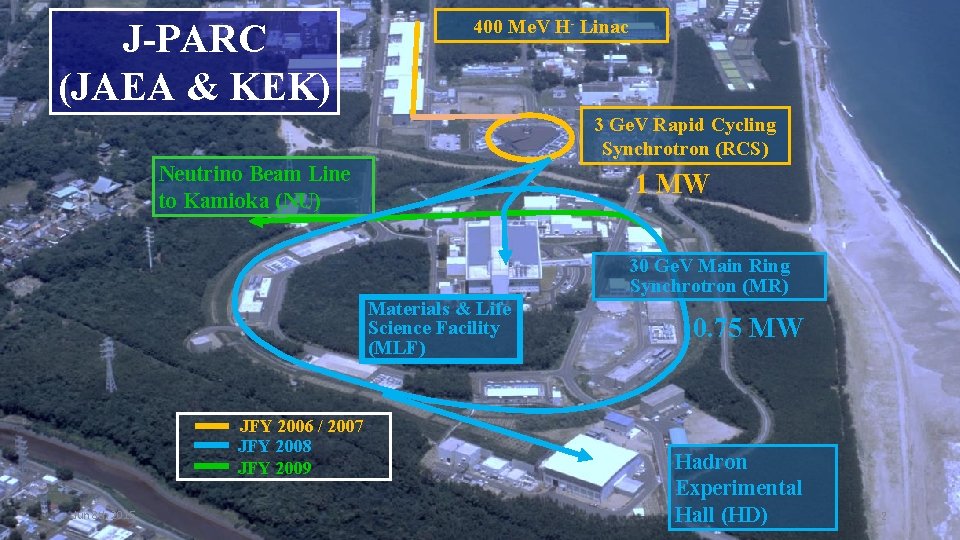
J-PARC (JAEA & KEK) 400 Me. V H- Linac 3 Ge. V Rapid Cycling Synchrotron (RCS) Neutrino Beam Line to Kamioka (NU) 1 MW 30 Ge. V Main Ring Synchrotron (MR) Materials & Life Science Facility (MLF) JFY 2006 / 2007 JFY 2008 JFY 2009 Jun 8 th 2015 0. 75 MW Hadron Experimental Hall (HD) 2
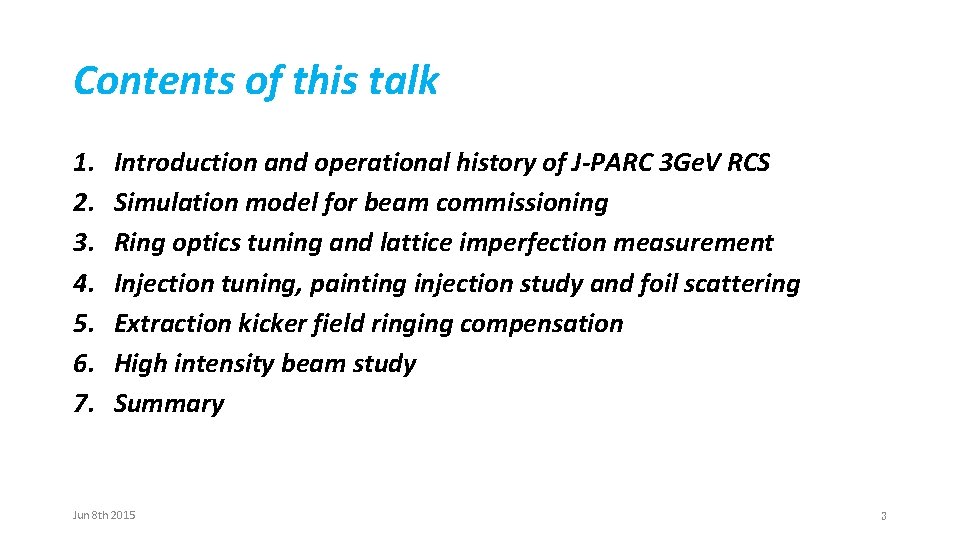
Contents of this talk 1. 2. 3. 4. 5. 6. 7. Introduction and operational history of J-PARC 3 Ge. V RCS Simulation model for beam commissioning Ring optics tuning and lattice imperfection measurement Injection tuning, painting injection study and foil scattering Extraction kicker field ringing compensation High intensity beam study Summary Jun 8 th 2015 3
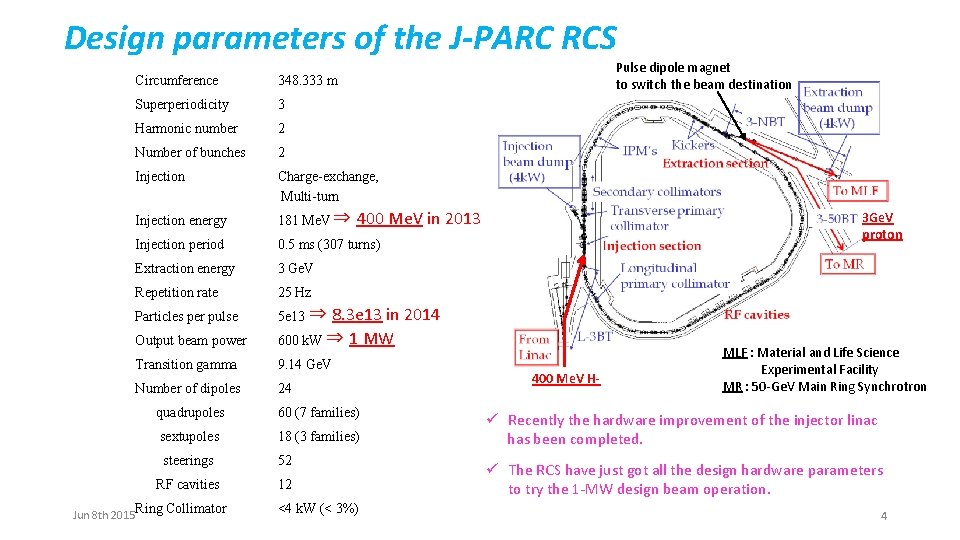
Design parameters of the J-PARC RCS Pulse dipole magnet to switch the beam destination Circumference 348. 333 m Superperiodicity 3 Harmonic number 2 Number of bunches 2 Injection Charge-exchange, Multi-turn Injection energy 181 Me. V ⇒ Injection period 0. 5 ms (307 turns) Extraction energy 3 Ge. V Repetition rate 25 Hz Particles per pulse 5 e 13 ⇒ 400 Me. V in 2013 Output beam power 8. 3 e 13 in 2014 600 k. W ⇒ 1 MW Transition gamma 9. 14 Ge. V Number of dipoles 24 quadrupoles 60 (7 families) sextupoles 18 (3 families) steerings 52 RF cavities 12 Ring Collimator Jun 8 th 2015 <4 k. W (< 3%) 3 Ge. V proton 400 Me. V H- MLF : Material and Life Science Experimental Facility MR : 50 -Ge. V Main Ring Synchrotron ü Recently the hardware improvement of the injector linac has been completed. ü The RCS have just got all the design hardware parameters to try the 1 -MW design beam operation. 4
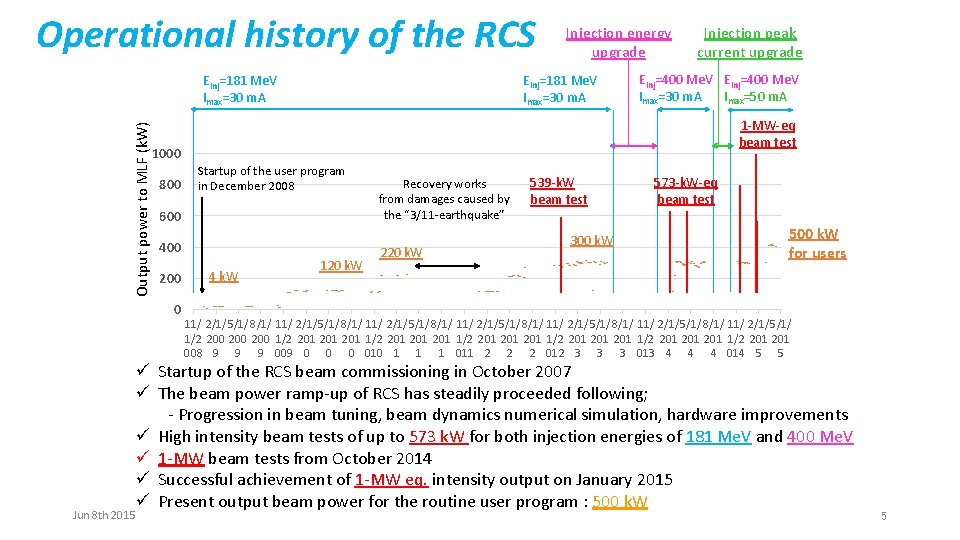
Operational history of the RCS Einj=181 Me. V Imax=30 m. A Output power to MLF (k. W) Injection energy upgrade Injection peak current upgrade Einj=400 Me. V Imax=30 m. A Imax=50 m. A 1 -MW-eq beam test 1000 800 Startup of the user program in December 2008 600 400 200 0 4 k. W 120 k. W Recovery works from damages caused by the “ 3/11 -earthquake” 220 k. W 539 -k. W beam test 300 k. W 573 -k. W-eq beam test 500 k. W for users 11/ 2/1/ 5/1/ 8/1/ 11/ 2/1/ 5/1/ 8/1/ 11/ 2/1/ 5/1/ 1/2 200 200 1/2 201 201 201 1/2 201 201 1/2 201 008 9 9 9 009 0 010 1 1 1 011 2 2 2 012 3 3 3 013 4 4 4 014 5 5 ü Startup of the RCS beam commissioning in October 2007 ü The beam power ramp-up of RCS has steadily proceeded following; - Progression in beam tuning, beam dynamics numerical simulation, hardware improvements ü High intensity beam tests of up to 573 k. W for both injection energies of 181 Me. V and 400 Me. V ü 1 -MW beam tests from October 2014 ü Successful achievement of 1 -MW eq. intensity output on January 2015 ü Present output beam power for the routine user program : 500 k. W Jun 8 th 2015 5
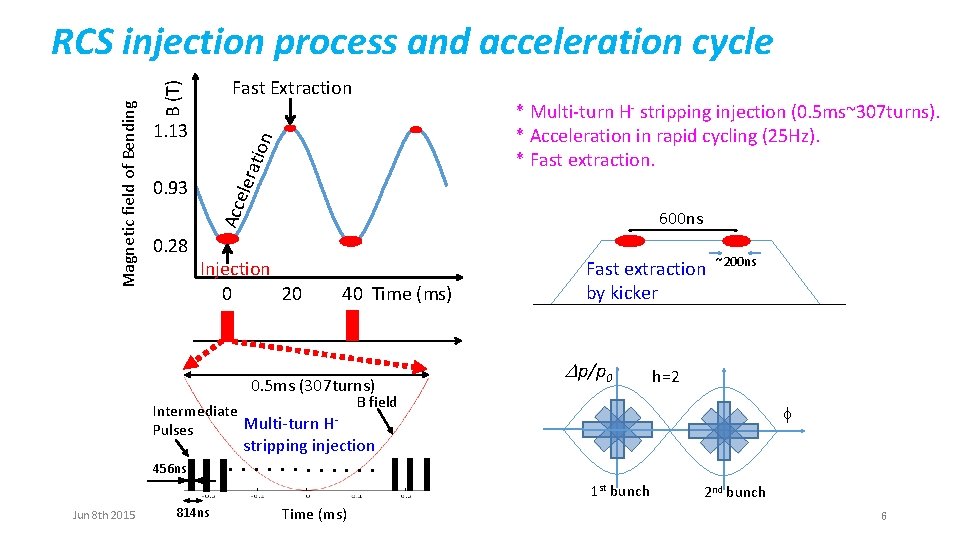
Fast Extraction B (T) eler a tion 1. 13 * Multi-turn H- stripping injection (0. 5 ms~307 turns). * Acceleration in rapid cycling (25 Hz). * Fast extraction. 0. 93 Acc Magnetic field of Bending RCS injection process and acceleration cycle 0. 28 600 ns Injection 0 20 40 Time (ms) 0. 5 ms (307 turns) Intermediate Multi-turn HPulses 456 ns Fast extraction by kicker Dp/p 0 814 ns h=2 B field f stripping injection. . . 1 st bunch Jun 8 th 2015 ~200 ns Time (ms) 2 nd bunch 6
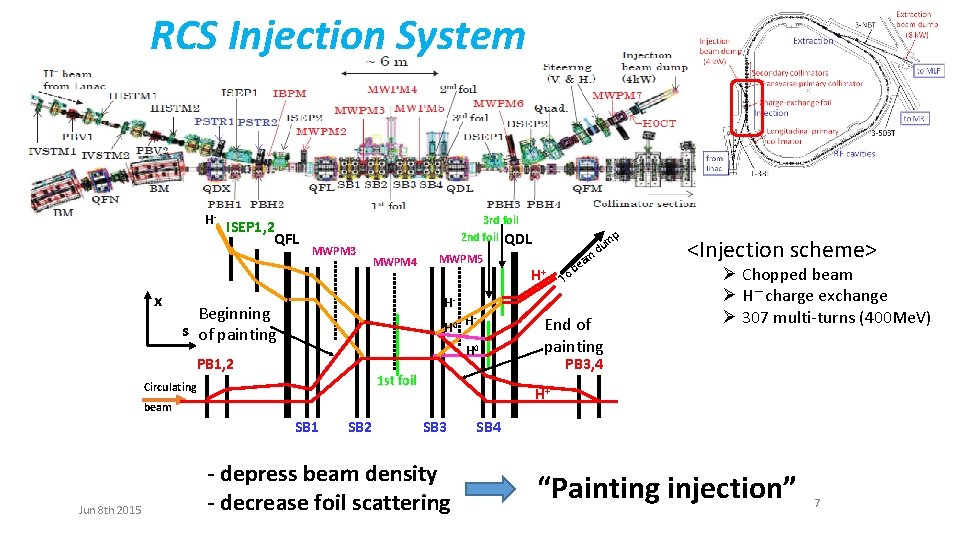
RCS Injection System H- x ISEP 1, 2 QFL MWPM 3 3 rd foil 2 nd foil QDL MWPM 4 MWPM 5 m H+ To a be H- Beginning s of painting H 0 HH 0 PB 1, 2 1 st foil Circulating SB 1 SB 2 End of painting <Injection scheme> Ø Chopped beam Ø H-charge exchange Ø 307 multi-turns (400 Me. V) PB 3, 4 H+ beam Jun 8 th 2015 p m du SB 3 - depress beam density - decrease foil scattering SB 4 “Painting injection” 7
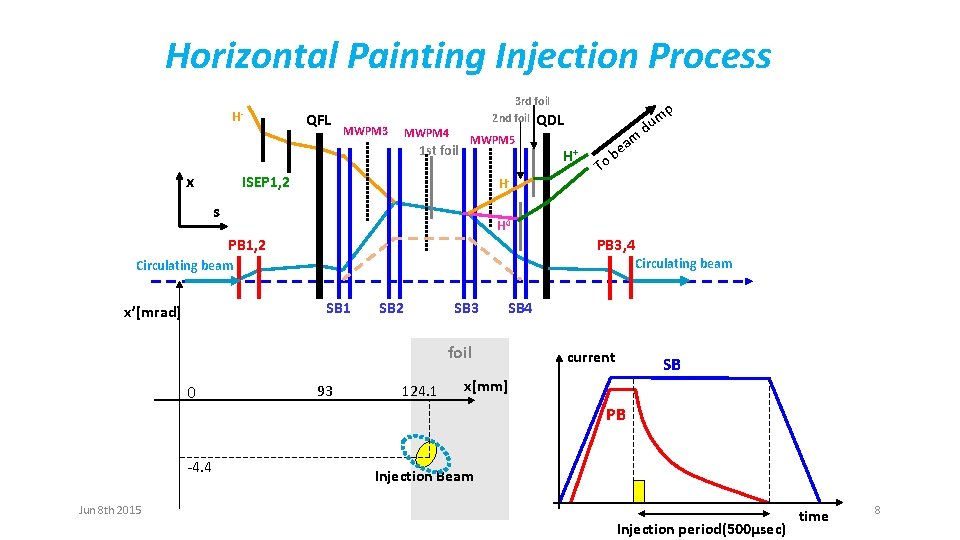
Horizontal Painting Injection Process H- QFL MWPM 3 3 rd foil 2 nd foil QDL MWPM 4 1 st foil x MWPM 5 ISEP 1, 2 H- s H+ e b o T PB 3, 4 Circulating beam SB 1 SB 2 SB 3 SB 4 foil 0 d H 0 PB 1, 2 x’[mrad] am p um 93 124. 1 current SB x[mm] PB -4. 4 Injection Beam Jun 8 th 2015 Injection period(500μsec) time 8
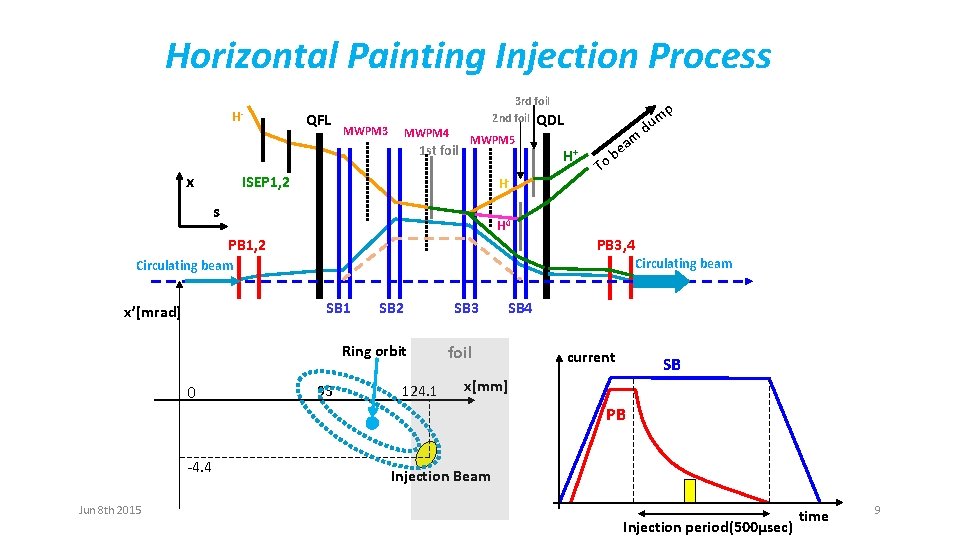
Horizontal Painting Injection Process H- QFL MWPM 3 3 rd foil 2 nd foil QDL MWPM 4 1 st foil x MWPM 5 ISEP 1, 2 H- s H+ am e b o T PB 3, 4 Circulating beam SB 1 SB 2 Ring orbit 0 d H 0 PB 1, 2 x’[mrad] p um 93 124. 1 SB 3 SB 4 foil current SB x[mm] PB -4. 4 Jun 8 th 2015 Injection Beam Injection period(500μsec) time 9
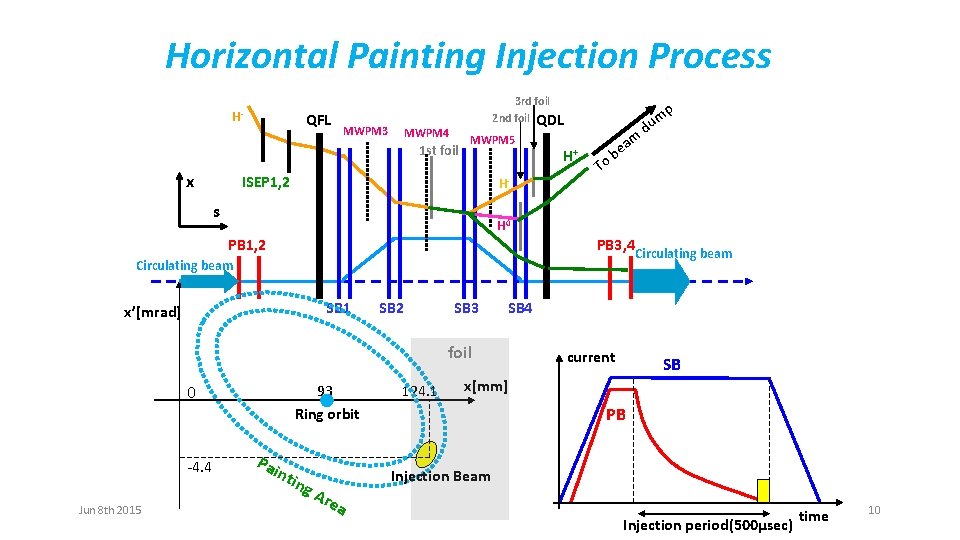
Horizontal Painting Injection Process H- QFL MWPM 3 3 rd foil 2 nd foil QDL MWPM 4 1 st foil x MWPM 5 ISEP 1, 2 H- s H+ am e b o T PB 3, 4 Circulating beam SB 1 x’[mrad] SB 2 SB 3 SB 4 foil 0 Jun 8 th 2015 d H 0 PB 1, 2 -4. 4 p um 93 Ring orbit Pa int ing 124. 1 current SB x[mm] PB Are Injection Beam a Injection period(500μsec) time 10
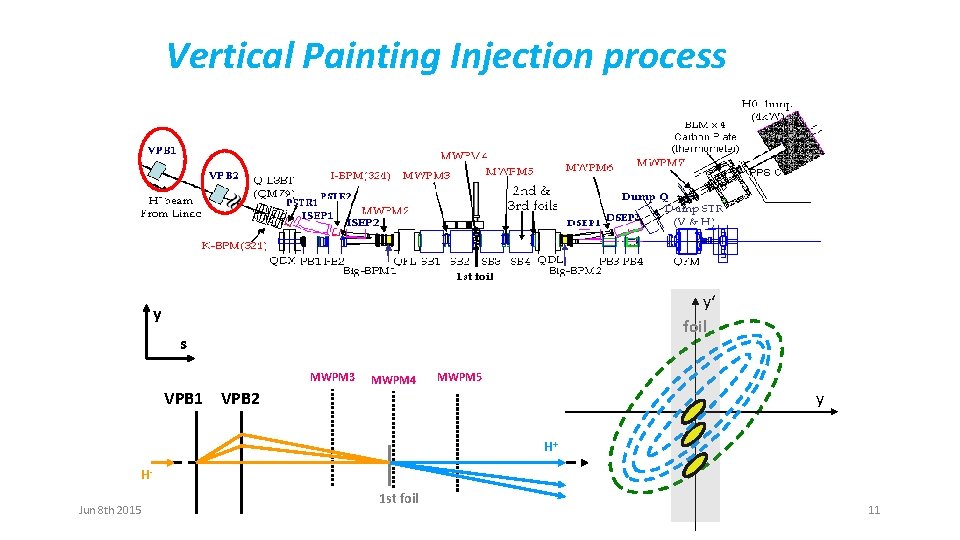
Vertical Painting Injection process y‘ foil y s MWPM 3 VPB 1 VPB 2 MWPM 4 MWPM 5 y H+ HJun 8 th 2015 1 st foil 11
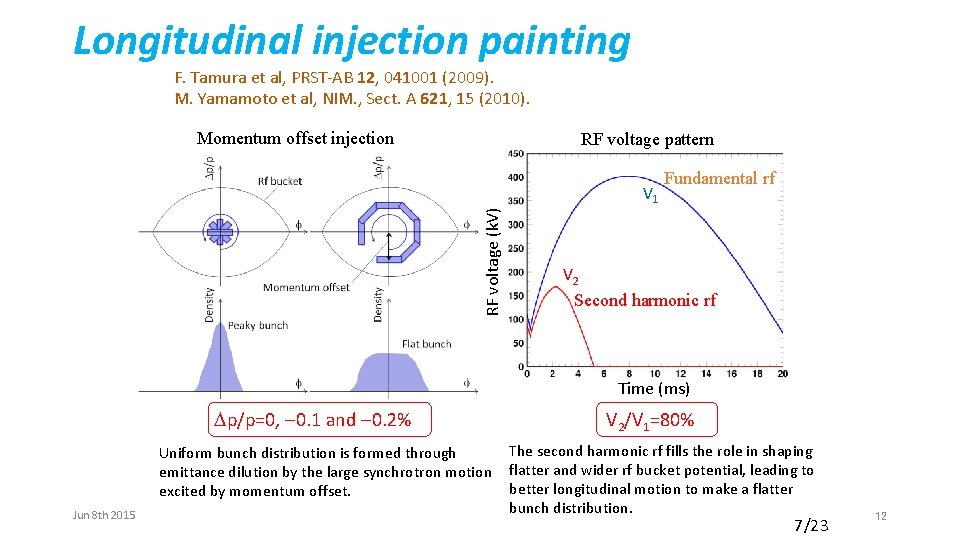
Longitudinal injection painting F. Tamura et al, PRST-AB 12, 041001 (2009). M. Yamamoto et al, NIM. , Sect. A 621, 15 (2010). Momentum offset injection RF voltage pattern RF voltage (k. V) V 1 Fundamental rf V 2 Second harmonic rf Time (ms) Dp/p=0, -0. 1 and -0. 2% Uniform bunch distribution is formed through emittance dilution by the large synchrotron motion excited by momentum offset. Jun 8 th 2015 V 2/V 1=80% The second harmonic rf fills the role in shaping flatter and wider rf bucket potential, leading to better longitudinal motion to make a flatter bunch distribution. 7/23 12
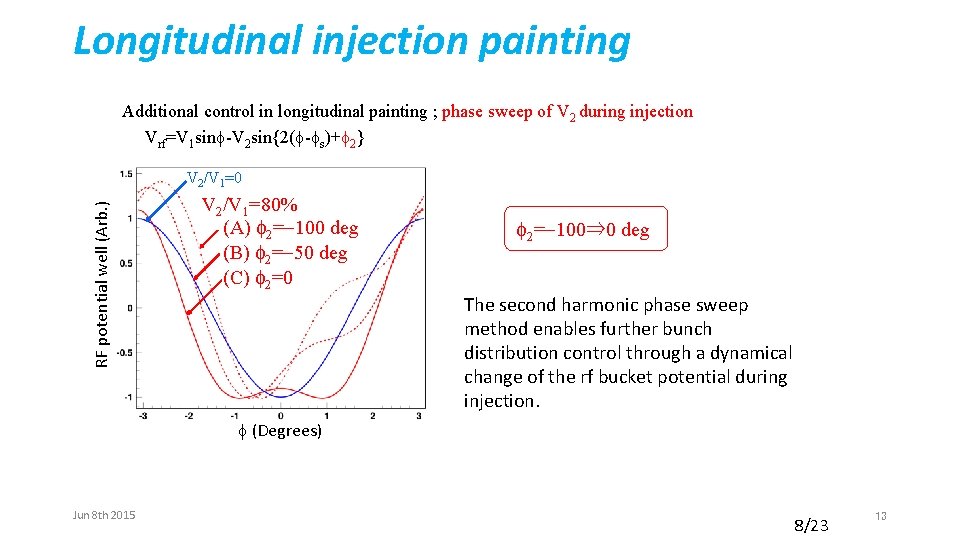
Longitudinal injection painting Additional control in longitudinal painting ; phase sweep of V 2 during injection Vrf=V 1 sinf-V 2 sin{2(f-fs)+f 2} RF potential well (Arb. ) V 2/V 1=0 V 2/V 1=80% (A) f 2=-100 deg (B) f 2=-50 deg (C) f 2=0 f 2=-100⇒ 0 deg The second harmonic phase sweep method enables further bunch distribution control through a dynamical change of the rf bucket potential during injection. f (Degrees) Jun 8 th 2015 8/23 13
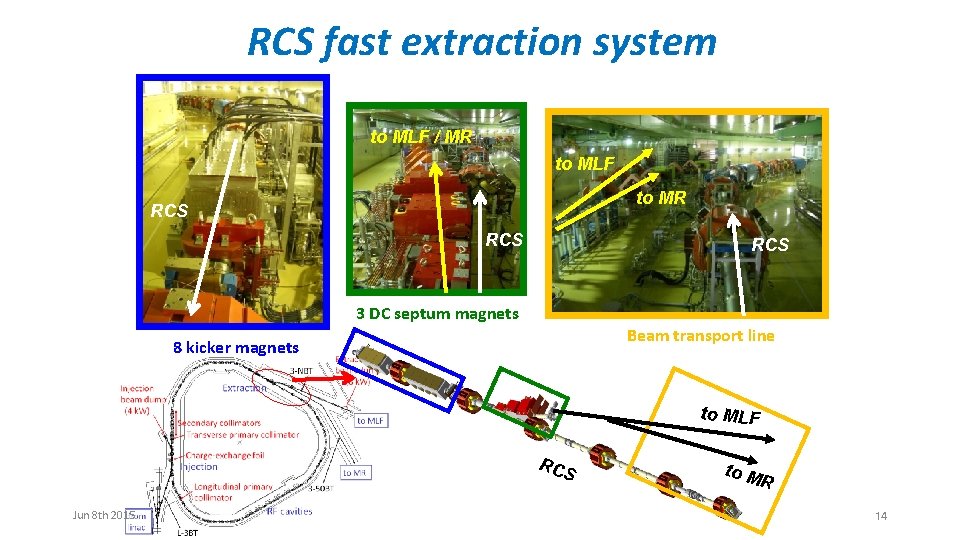
RCS fast extraction system to MLF / MR to MLF to MR RCS RCS 3 DC septum magnets Beam transport line 8 kicker magnets to MLF RCS Jun 8 th 2015 to M R 14
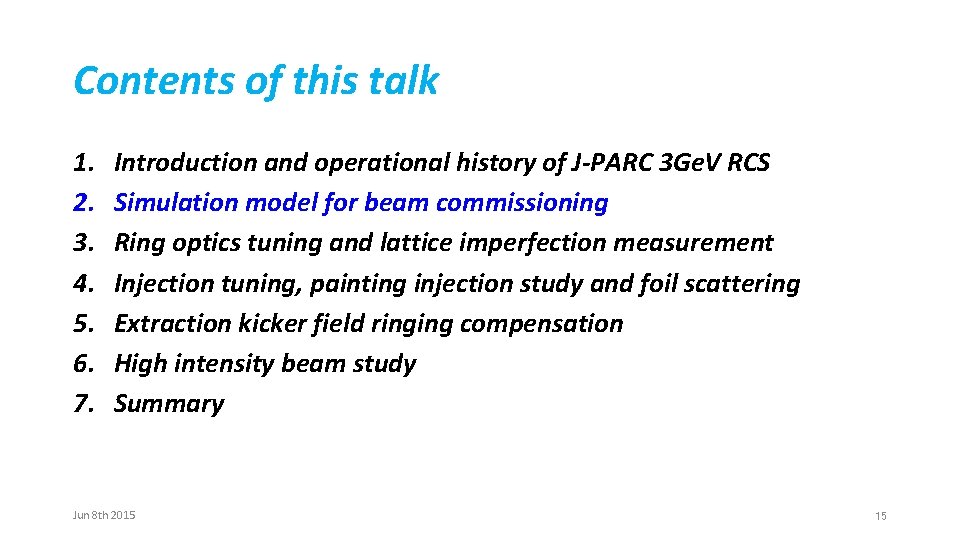
Contents of this talk 1. 2. 3. 4. 5. 6. 7. Introduction and operational history of J-PARC 3 Ge. V RCS Simulation model for beam commissioning Ring optics tuning and lattice imperfection measurement Injection tuning, painting injection study and foil scattering Extraction kicker field ringing compensation High intensity beam study Summary Jun 8 th 2015 15
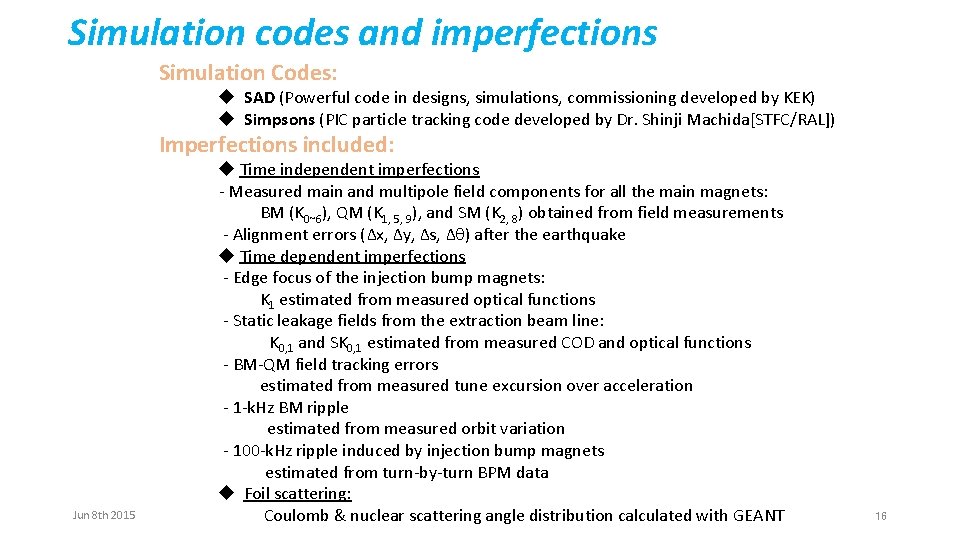
Simulation codes and imperfections Simulation Codes: u SAD (Powerful code in designs, simulations, commissioning developed by KEK) u Simpsons (PIC particle tracking code developed by Dr. Shinji Machida[STFC/RAL]) Imperfections included: Jun 8 th 2015 u Time independent imperfections - Measured main and multipole field components for all the main magnets: BM (K 0~6), QM (K 1, 5, 9), and SM (K 2, 8) obtained from field measurements - Alignment errors (Δx, Δy, Δs, Δq) after the earthquake u Time dependent imperfections - Edge focus of the injection bump magnets: K 1 estimated from measured optical functions - Static leakage fields from the extraction beam line: K 0, 1 and SK 0, 1 estimated from measured COD and optical functions - BM-QM field tracking errors estimated from measured tune excursion over acceleration - 1 -k. Hz BM ripple estimated from measured orbit variation - 100 -k. Hz ripple induced by injection bump magnets estimated from turn-by-turn BPM data u Foil scattering: Coulomb & nuclear scattering angle distribution calculated with GEANT 16
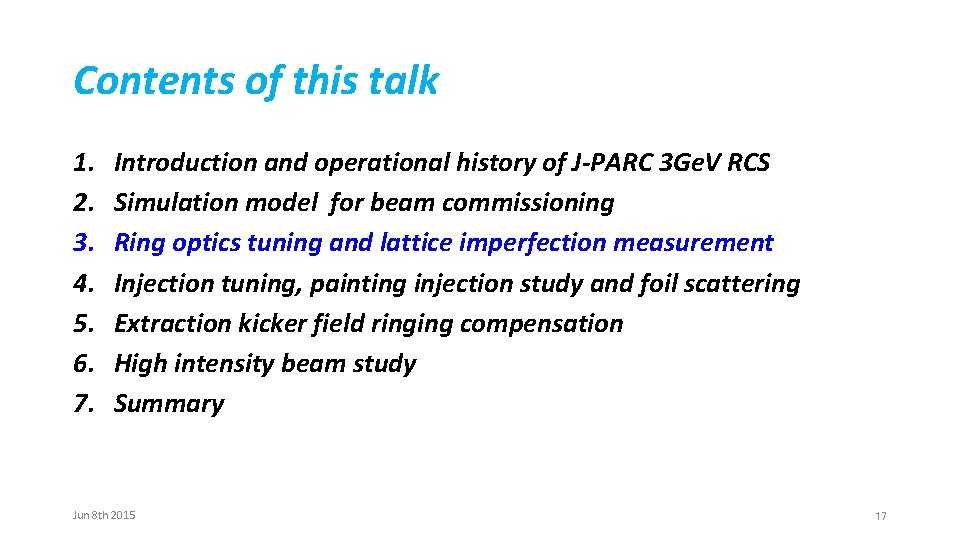
Contents of this talk 1. 2. 3. 4. 5. 6. 7. Introduction and operational history of J-PARC 3 Ge. V RCS Simulation model for beam commissioning Ring optics tuning and lattice imperfection measurement Injection tuning, painting injection study and foil scattering Extraction kicker field ringing compensation High intensity beam study Summary Jun 8 th 2015 17
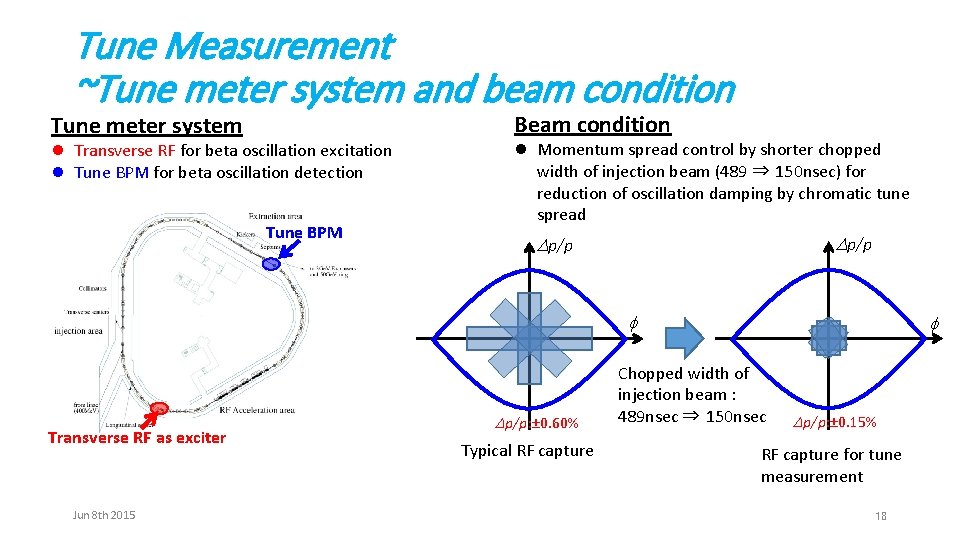
Tune Measurement ~Tune meter system and beam condition Beam condition Tune meter system l Transverse RF for beta oscillation excitation l Tune BPM for beta oscillation detection Tune BPM l Momentum spread control by shorter chopped width of injection beam (489 ⇒ 150 nsec) for reduction of oscillation damping by chromatic tune spread Dp/p f Transverse RF as exciter Jun 8 th 2015 Dp/p: ± 0. 60% Typical RF capture f Chopped width of injection beam : 489 nsec ⇒ 150 nsec Dp/p: ± 0. 15% RF capture for tune measurement 18
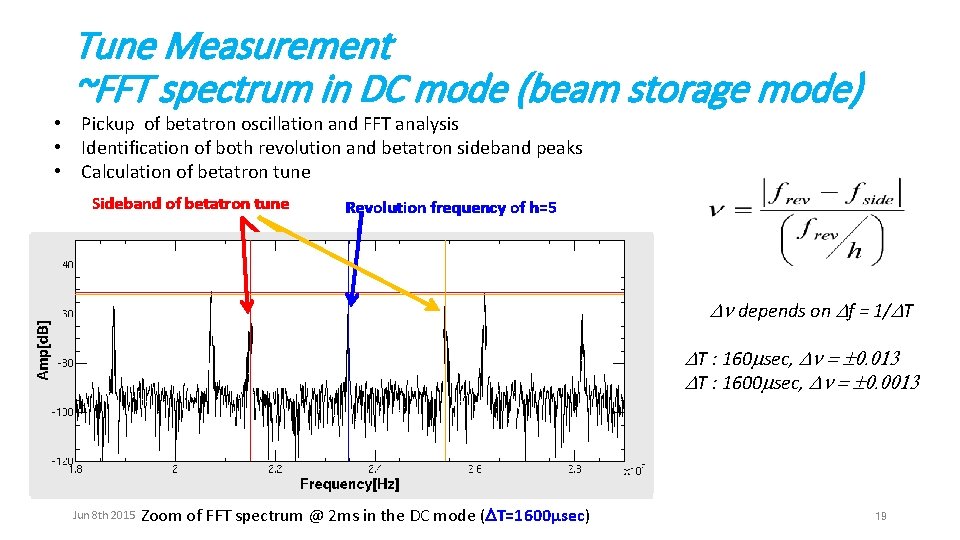
Tune Measurement ~FFT spectrum in DC mode (beam storage mode) • Pickup of betatron oscillation and FFT analysis • Identification of both revolution and betatron sideband peaks • Calculation of betatron tune Sideband of betatron tune Revolution frequency of h=5 Dn depends on Df = 1/DT DT : 160 msec, Dn = ± 0. 013 DT : 1600 msec, Dn = ± 0. 0013 Jun 8 th 2015 FFT spectrum @ 2 ms@ in 2 ms the DC mode Zoom of FFT spectrum in the DC(DT=160μsec) mode (DT=1600μsec) (DT=160μsec) 19
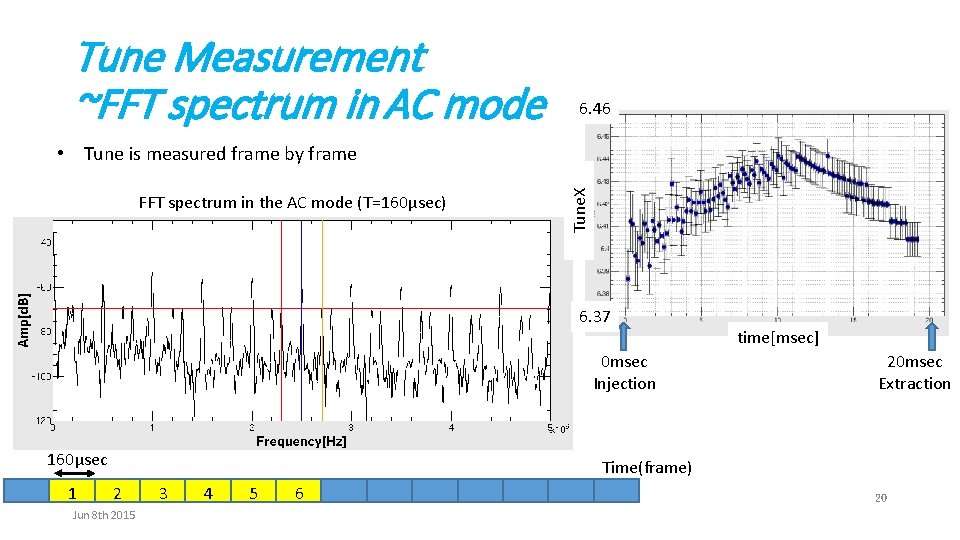
Tune Measurement ~FFT spectrum in AC mode 6. 46 FFT spectrum in the AC mode (T=160μsec) Tune. X • Tune is measured frame by frame 6. 37 0 msec Injection 160μsec 1 time[msec] 20 msec Extraction Time(frame) 2 Jun 8 th 2015 3 4 5 6 20
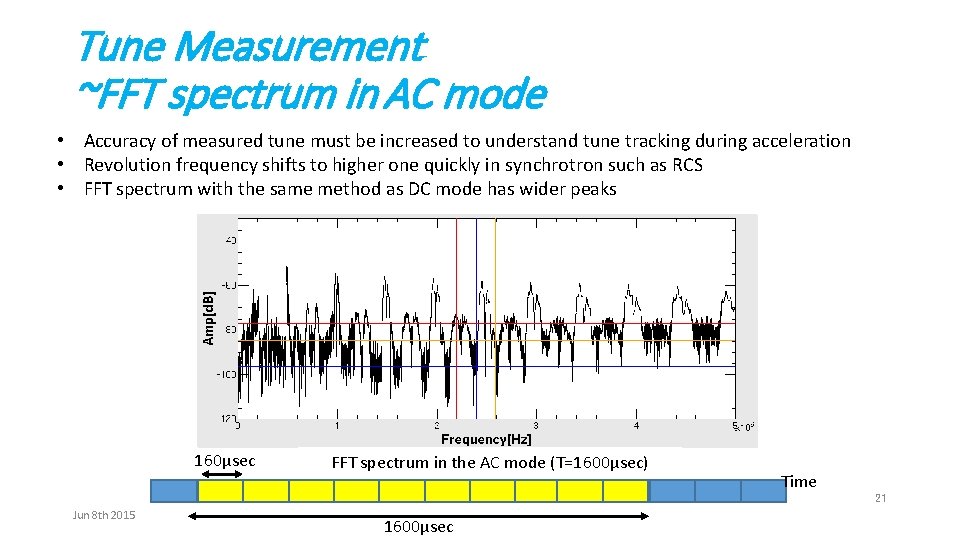
Tune Measurement ~FFT spectrum in AC mode • Accuracy of measured tune must be increased to understand tune tracking during acceleration • Revolution frequency shifts to higher one quickly in synchrotron such as RCS • FFT spectrum with the same method as DC mode has wider peaks 160μsec FFTspectrumin inthe the. AC ACmode(T=1600μsec) (T=160μsec) FFT 1 Jun 8 th 2015 1600μsec Time 21
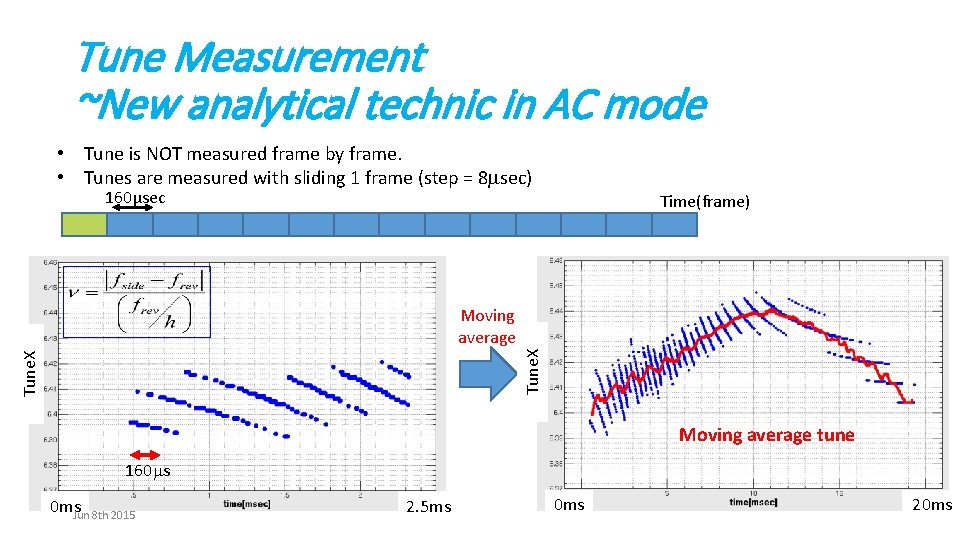
Tune Measurement ~New analytical technic in AC mode • Tune is NOT measured frame by frame. • Tunes are measured with sliding 1 frame (step = 8 msec) 160μsec Time(frame) Tune. X Moving average tune 160 ms 0 ms. Jun 8 th 2015 2. 5 ms 0 ms 22 20 ms
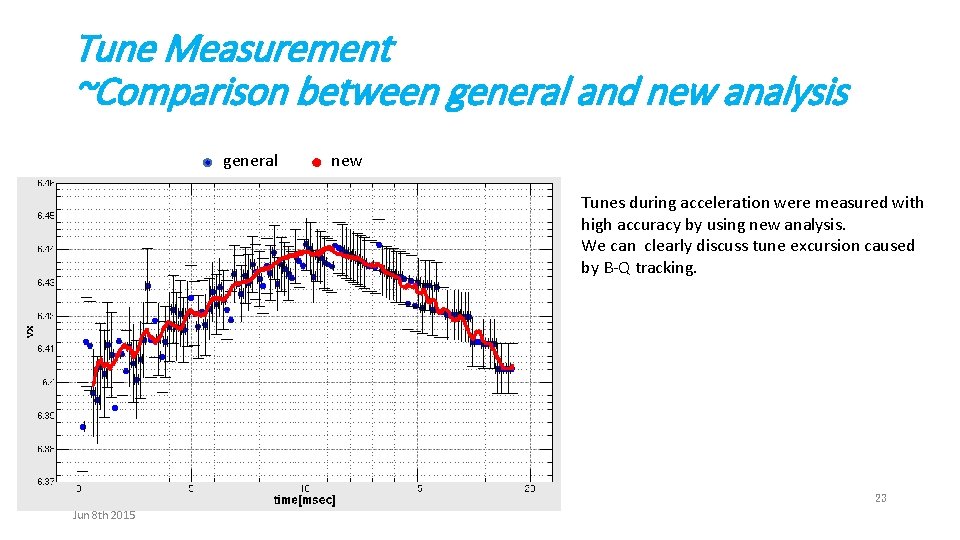
Tune Measurement ~Comparison between general and new analysis general new Tunes during acceleration were measured with high accuracy by using new analysis. We can clearly discuss tune excursion caused by B-Q tracking. 23 Jun 8 th 2015
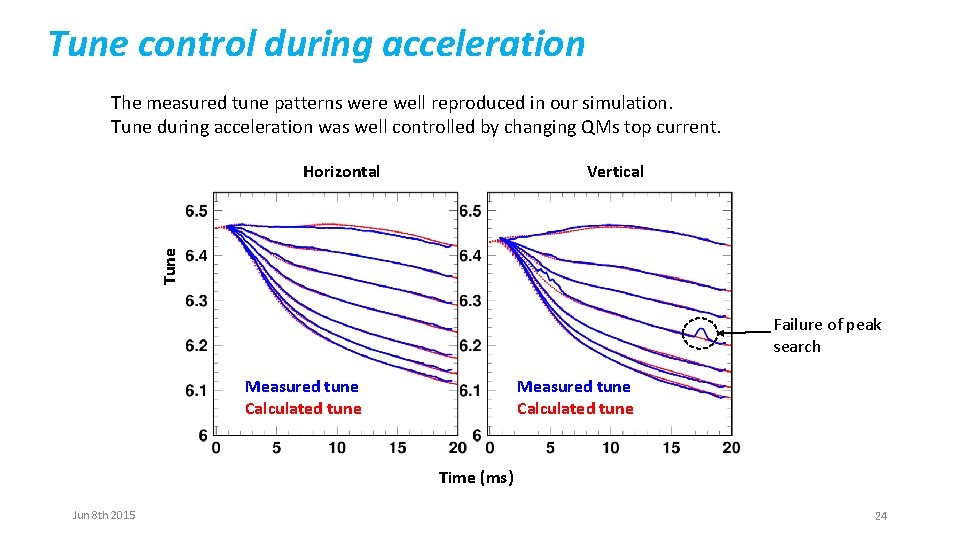
Tune control during acceleration The measured tune patterns were well reproduced in our simulation. Tune during acceleration was well controlled by changing QMs top current. Vertical Tune Horizontal Failure of peak search Measured tune Calculated tune Time (ms) Jun 8 th 2015 24
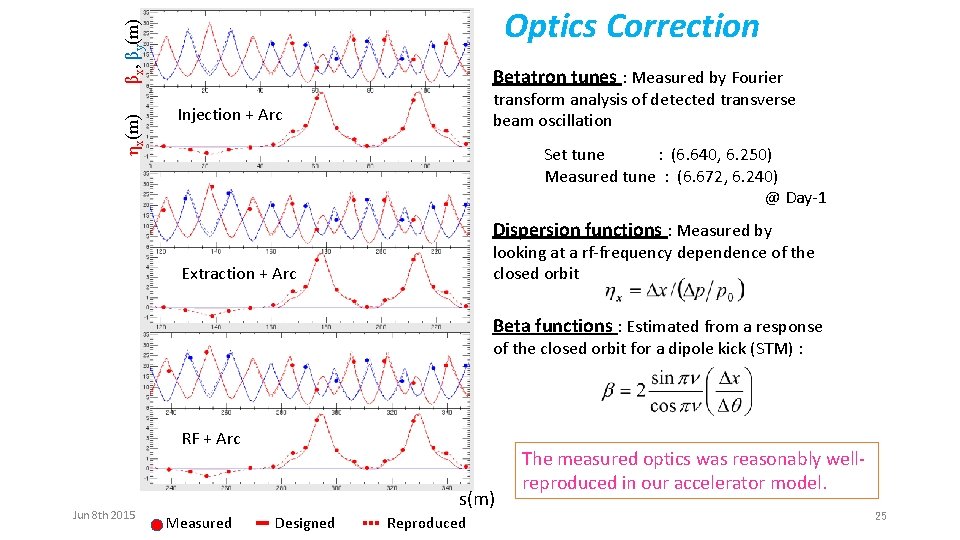
ηx(m) βx, βy(m) Optics Correction Betatron tunes : Measured by Fourier transform analysis of detected transverse beam oscillation Injection + Arc Set tune : (6. 640, 6. 250) Measured tune : (6. 672, 6. 240) @ Day-1 Dispersion functions : Measured by looking at a rf-frequency dependence of the closed orbit Extraction + Arc Beta functions : Estimated from a response of the closed orbit for a dipole kick (STM) : RF + Arc Jun 8 th 2015 s(m) Measured Designed Reproduced The measured optics was reasonably wellreproduced in our accelerator model. 25
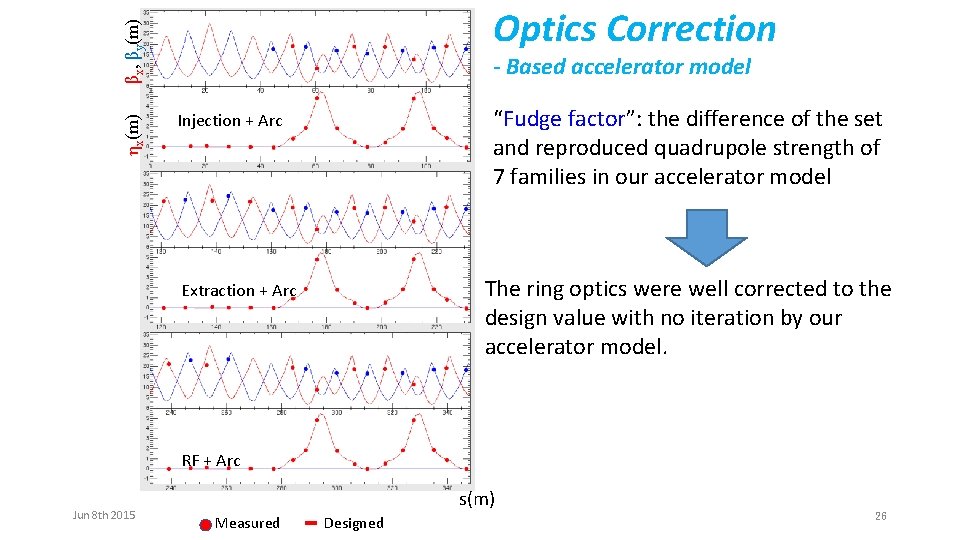
ηx(m) βx, βy(m) Optics Correction - Based accelerator model “Fudge factor”: the difference of the set and reproduced quadrupole strength of 7 families in our accelerator model Injection + Arc The ring optics were well corrected to the design value with no iteration by our accelerator model. Extraction + Arc RF + Arc Jun 8 th 2015 s(m) Measured Designed 26
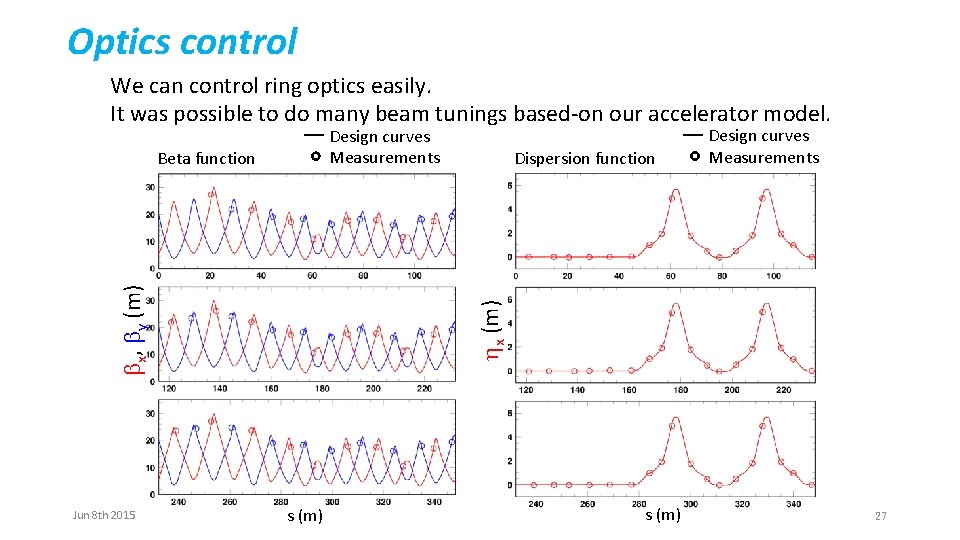
Optics control We can control ring optics easily. It was possible to do many beam tunings based-on our accelerator model. Design curves Measurements Jun 8 th 2015 Dispersion function hx (m) bx, by (m) Beta function Design curves Measurements s (m) 27
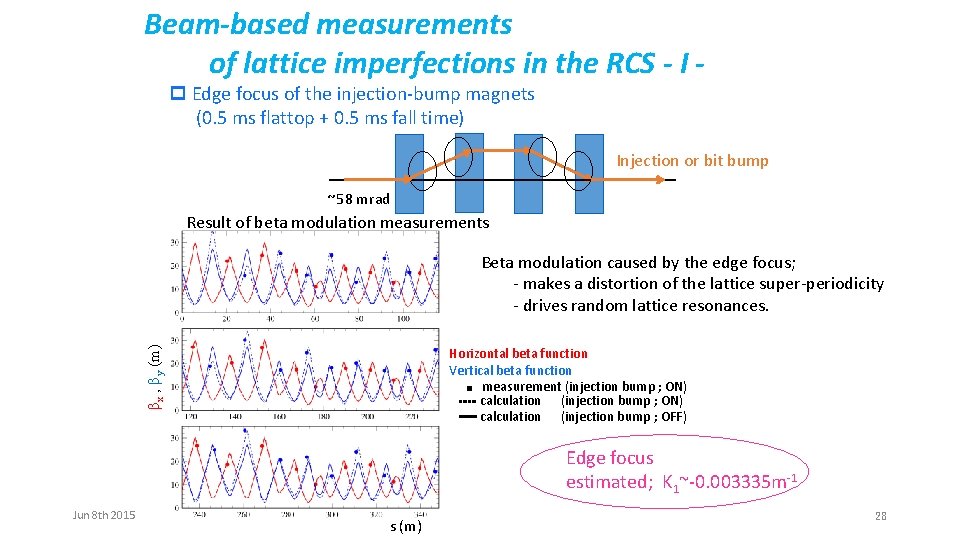
Beam-based measurements of lattice imperfections in the RCS - I p Edge focus of the injection-bump magnets (0. 5 ms flattop + 0. 5 ms fall time) Injection or bit bump ~58 mrad Result of beta modulation measurements βx , βy (m) Beta modulation caused by the edge focus; - makes a distortion of the lattice super-periodicity - drives random lattice resonances. Horizontal beta function Vertical beta function ● measurement (injection bump ; ON) calculation (injection bump ; OFF) Edge focus estimated; K 1~-0. 003335 m-1 Jun 8 th 2015 s (m) 28
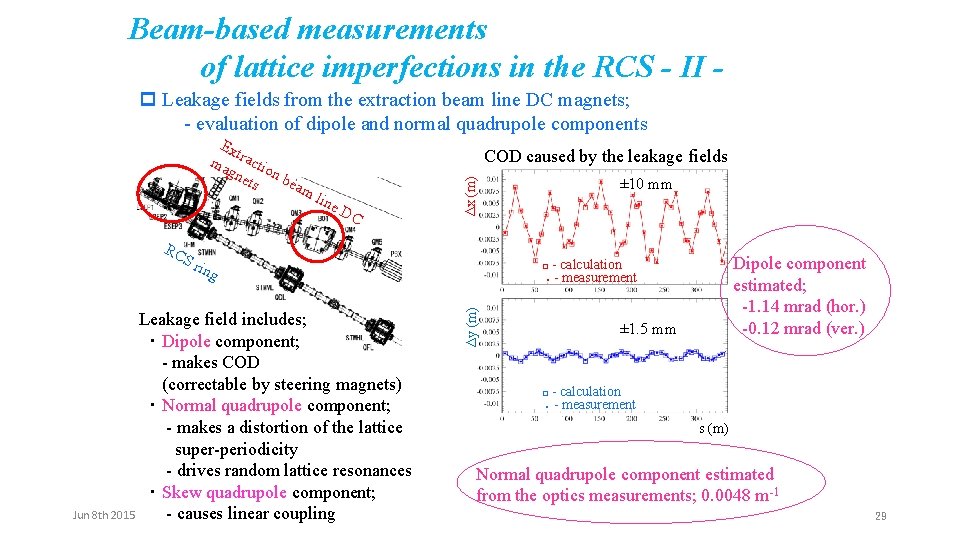
Beam-based measurements of lattice imperfections in the RCS - II p Leakage fields from the extraction beam line DC magnets; - evaluation of dipole and normal quadrupole components lin e DC Sr ing Leakage field includes; ・ Dipole component; - makes COD (correctable by steering magnets) ・ Normal quadrupole component; - makes a distortion of the lattice super-periodicity - drives random lattice resonances ・ Skew quadrupole component; Jun 8 th 2015 - causes linear coupling ± 10 mm □ ● Δy (m) RC COD caused by the leakage fields Δx (m) Ex t ma ractio gne n b ts eam Dipole component estimated; -1. 14 mrad (hor. ) -0. 12 mrad (ver. ) - calculation - measurement ± 1. 5 mm □ ● - calculation - measurement s (m) Normal quadrupole component estimated from the optics measurements; 0. 0048 m-1 29
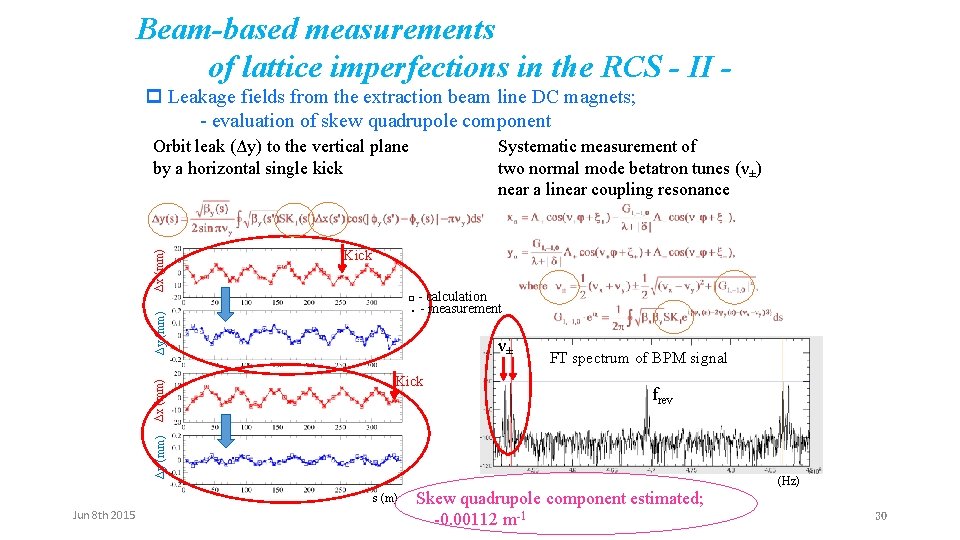
Beam-based measurements of lattice imperfections in the RCS - II p Leakage fields from the extraction beam line DC magnets; - evaluation of skew quadrupole component Systematic measurement of two normal mode betatron tunes (ν±) near a linear coupling resonance Kick □ ● - calculation - measurement ν± Kick FT spectrum of BPM signal frev Δy (mm) Δx (mm) Orbit leak (Δy) to the vertical plane by a horizontal single kick (Hz) s (m) Jun 8 th 2015 Skew quadrupole component estimated; -0. 00112 m-1 30
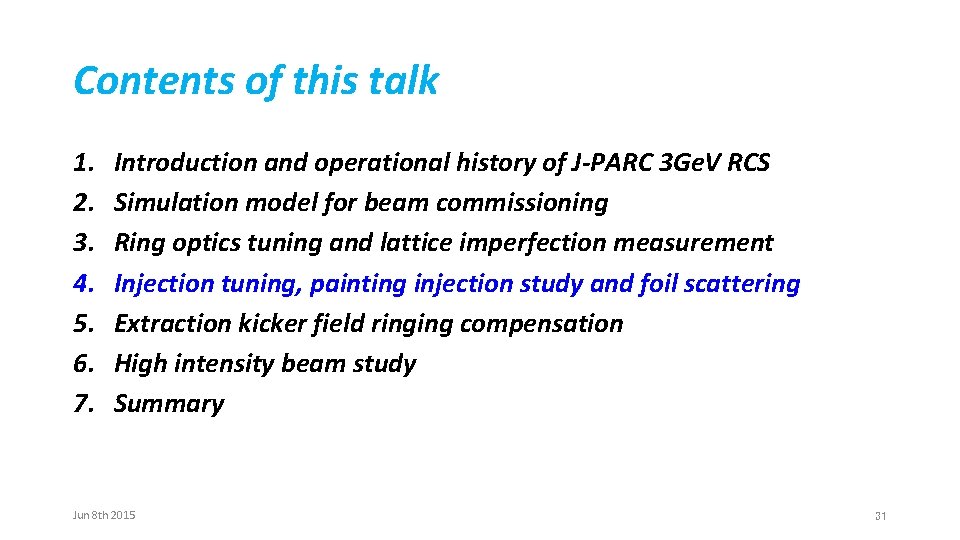
Contents of this talk 1. 2. 3. 4. 5. 6. 7. Introduction and operational history of J-PARC 3 Ge. V RCS Simulation model for beam commissioning Ring optics tuning and lattice imperfection measurement Injection tuning, painting injection study and foil scattering Extraction kicker field ringing compensation High intensity beam study Summary Jun 8 th 2015 31
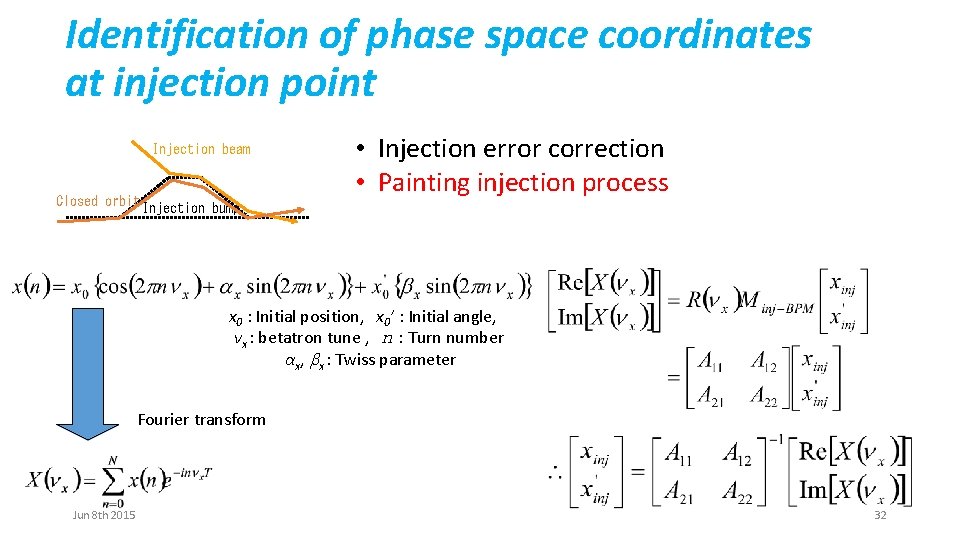
Identification of phase space coordinates at injection point Injection beam Closed orbit • Injection error correction • Painting injection process Injection bump x 0 : Initial position, x 0’ : Initial angle, νx : betatron tune , n : Turn number αx, bx : Twiss parameter Fourier transform Jun 8 th 2015 32
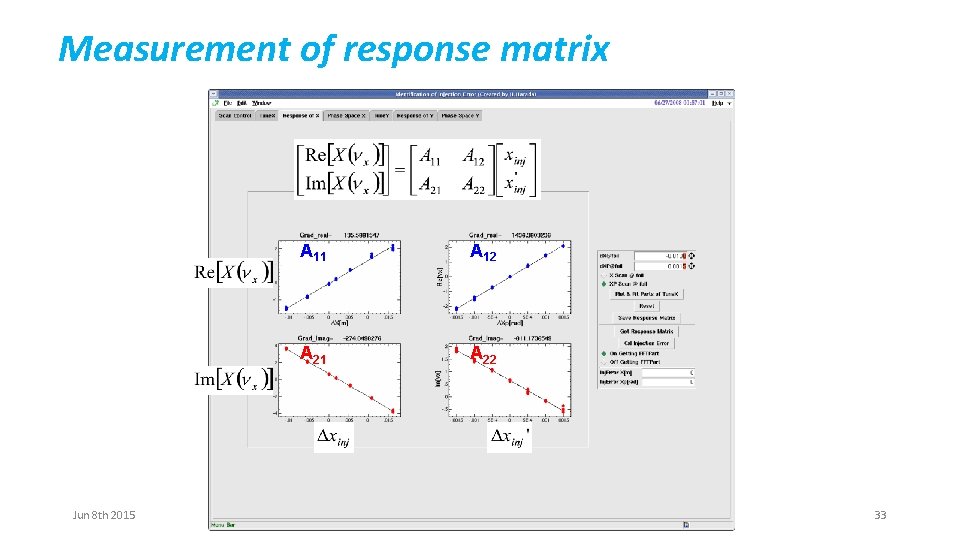
Measurement of response matrix A 11 A 12 Detected signals at left-side and right-side electrode A 21 Jun 8 th 2015 A 22 33
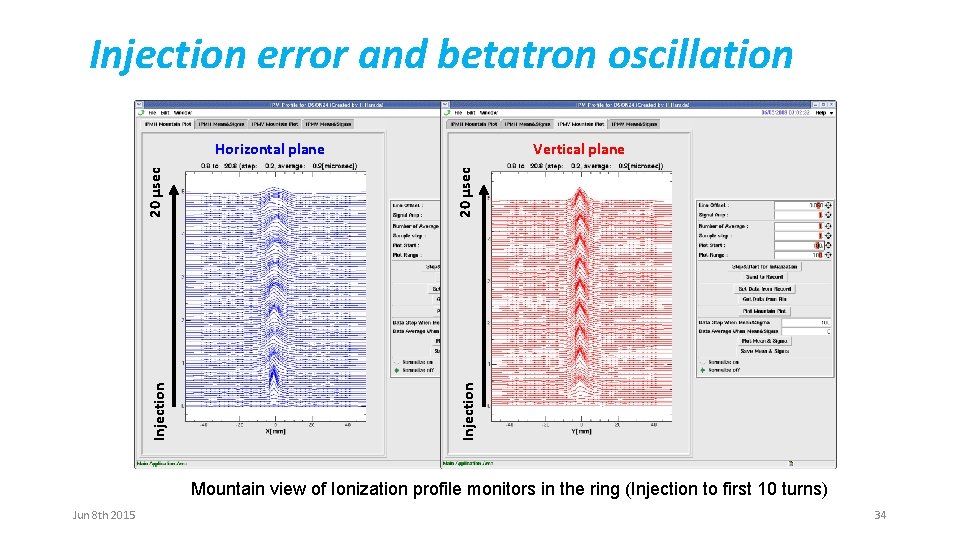
Injection error and betatron oscillation 20 μsec Vertical plane Injection 20 μsec Horizontal plane Mountain view of Ionization profile monitors in the ring (Injection to first 10 turns) Jun 8 th 2015 34
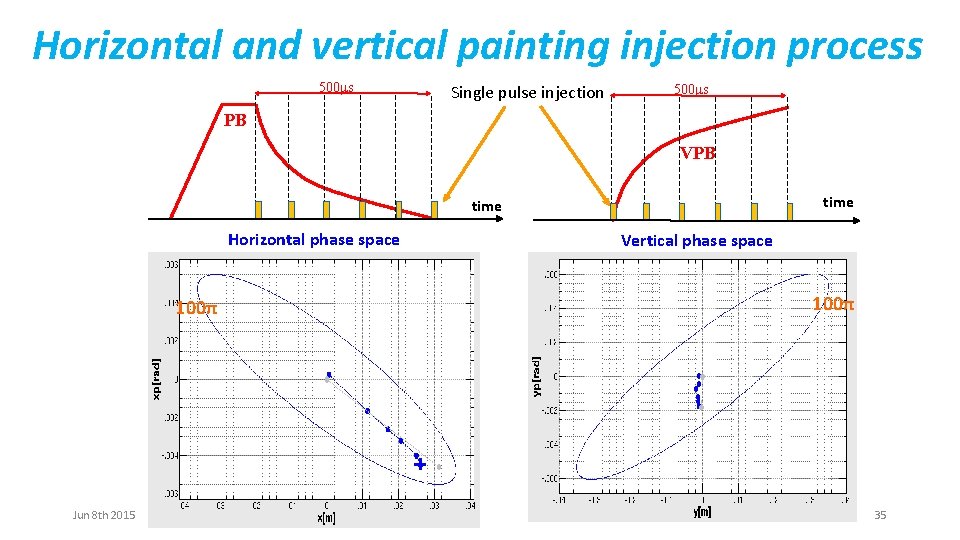
Horizontal and vertical painting injection process 500 ms Single pulse injection 500 ms PB VPB time Horizontal phase space 100π Jun 8 th 2015 Vertical phase space 100π 35
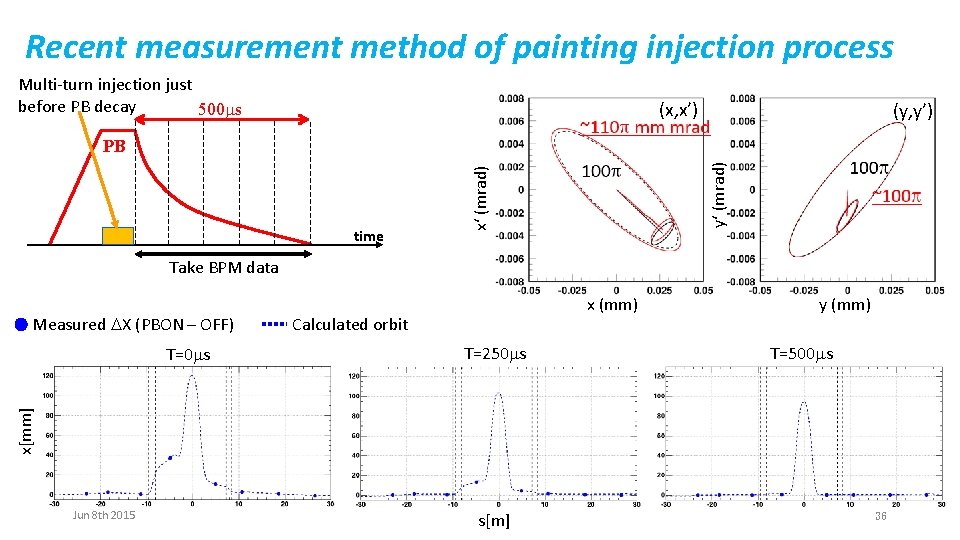
Recent measurement method of painting injection process Multi-turn injection just before PB decay 500 ms (x, x’) (y, y’) x‘ (mrad) time y‘ (mrad) PB Take BPM data Measured DX (PBON – OFF) Calculated orbit T=250 ms y (mm) T=500 ms x[mm] T=0 ms x (mm) Jun 8 th 2015 s[m] 36
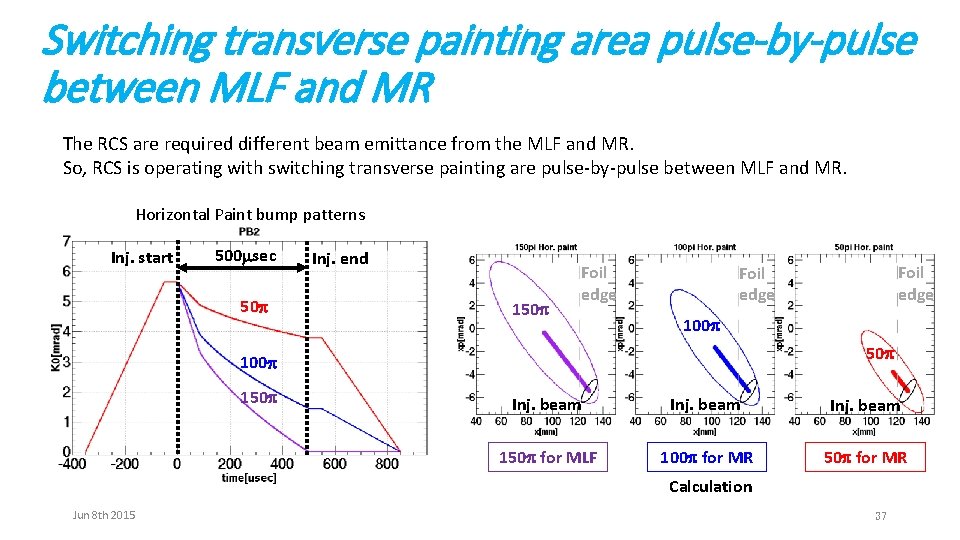
Switching transverse painting area pulse-by-pulse between MLF and MR The RCS are required different beam emittance from the MLF and MR. So, RCS is operating with switching transverse painting are pulse-by-pulse between MLF and MR. Horizontal Paint bump patterns Inj. start 500 msec 50 p Inj. end 150 p Foil edge 100 p 50 p 100 p 150 p Foil edge Inj. beam 150 p for MLF 100 p for MR 50 p for MR Calculation Jun 8 th 2015 37
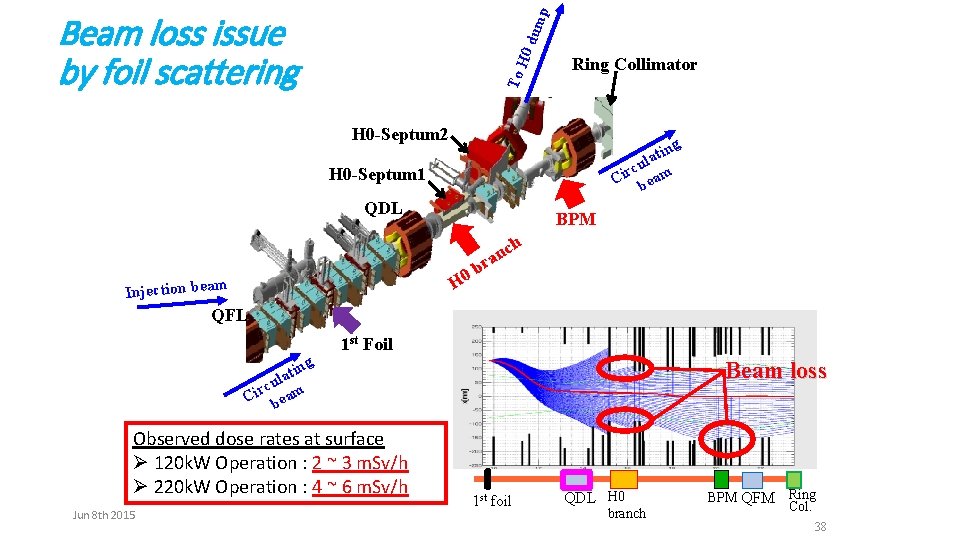
To H 0 dum p Beam loss issue by foil scattering Ring Collimator H 0 -Septum 2 g tin a l cu m r i C bea H 0 -Septum 1 QDL BPM c an r b 0 H Injection beam h QFL 1 st Foil g tin a l cu Cir beam Observed dose rates at surface Ø 120 k. W Operation : 2 ~ 3 m. Sv/h Ø 220 k. W Operation : 4 ~ 6 m. Sv/h Jun 8 th 2015 Beam loss 1 st foil QDL H 0 branch BPM QFM Ring Col. 38
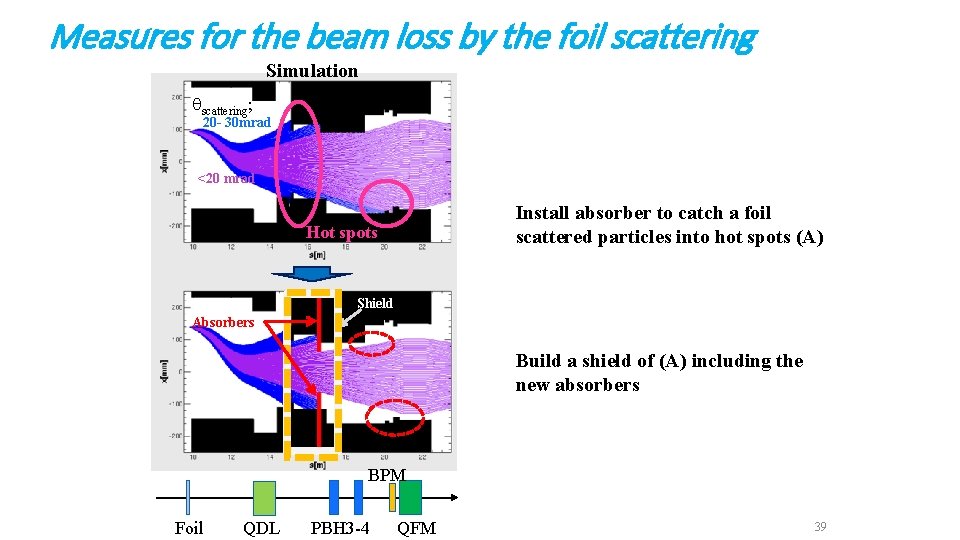
Measures for the beam loss by the foil scattering Simulation qscattering; 20 - 30 mrad <20 mrad Install absorber to catch a foil scattered particles into hot spots (A) Hot spots Shield Absorbers Build a shield of (A) including the new absorbers BPM Foil QDL PBH 3 -4 QFM 39
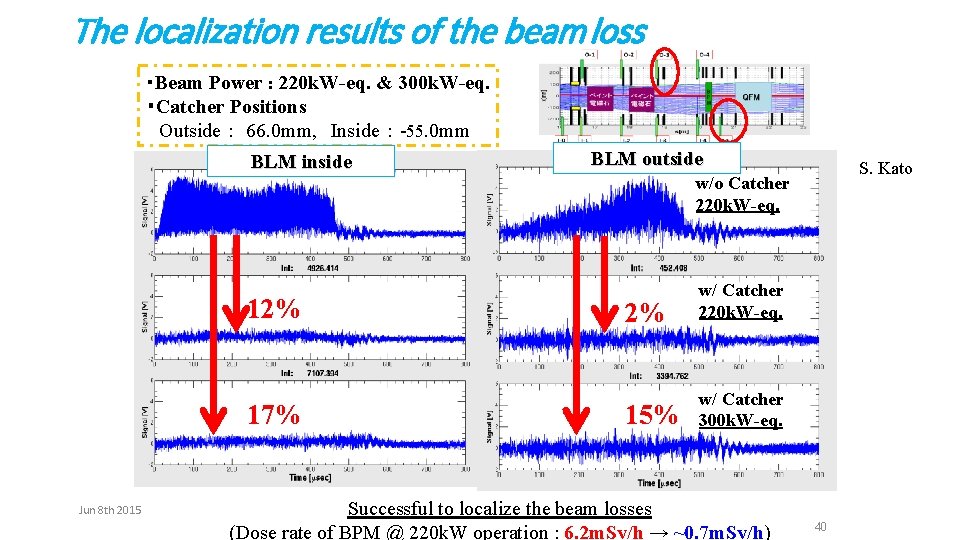
The localization results of the beam loss ・Beam Power : 220 k. W-eq. & 300 k. W-eq. ・Catcher Positions Outside : 66. 0 mm, Inside : -55. 0 mm BLM inside 12% 17% Jun 8 th 2015 BLM outside S. Kato w/o Catcher 220 k. W-eq. 2% w/ Catcher 220 k. W-eq. 15% w/ Catcher 300 k. W-eq. Successful to localize the beam losses (Dose rate of BPM @ 220 k. W operation : 6. 2 m. Sv/h → ~0. 7 m. Sv/h) 40
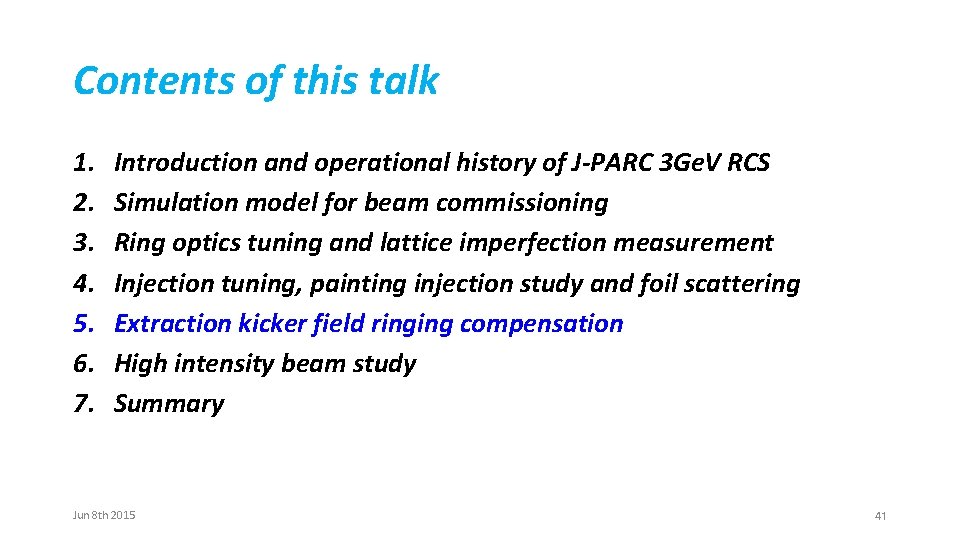
Contents of this talk 1. 2. 3. 4. 5. 6. 7. Introduction and operational history of J-PARC 3 Ge. V RCS Simulation model for beam commissioning Ring optics tuning and lattice imperfection measurement Injection tuning, painting injection study and foil scattering Extraction kicker field ringing compensation High intensity beam study Summary Jun 8 th 2015 41
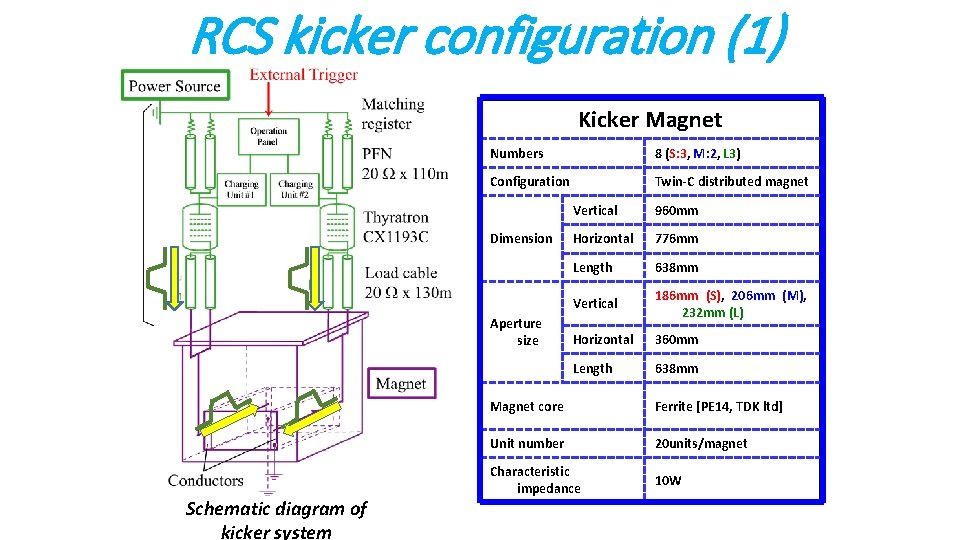
RCS kicker configuration (1) Kicker Magnet Numbers 8 (S: 3, M: 2, L 3) Configuration Twin-C distributed magnet Dimension Aperture size Schematic diagram of kicker system Vertical 960 mm Horizontal 776 mm Length 638 mm Vertical 186 mm (S), 206 mm (M), 232 mm (L) Horizontal 360 mm Length 638 mm Magnet core Ferrite [PE 14, TDK ltd] Unit number 20 units/magnet Characteristic impedance 10 W
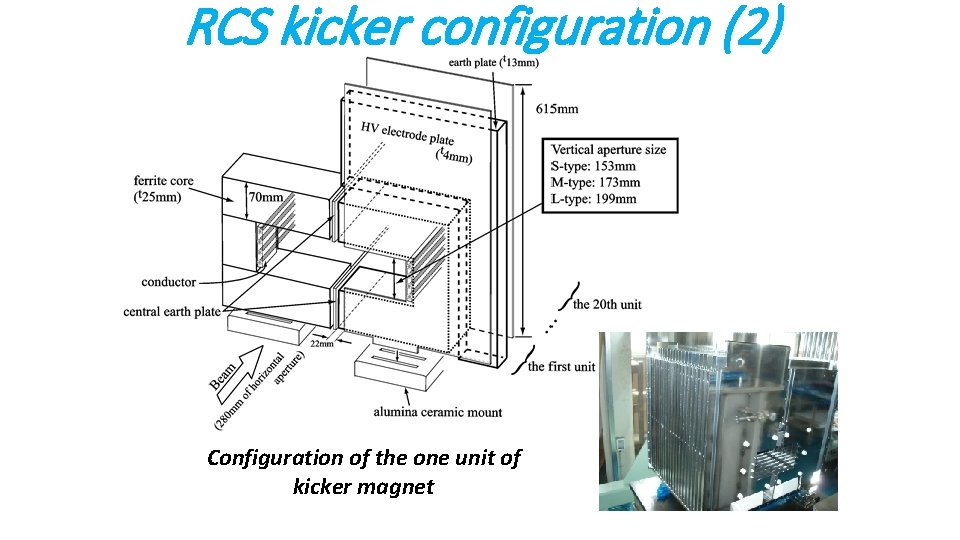
RCS kicker configuration (2) Configuration of the one unit of kicker magnet
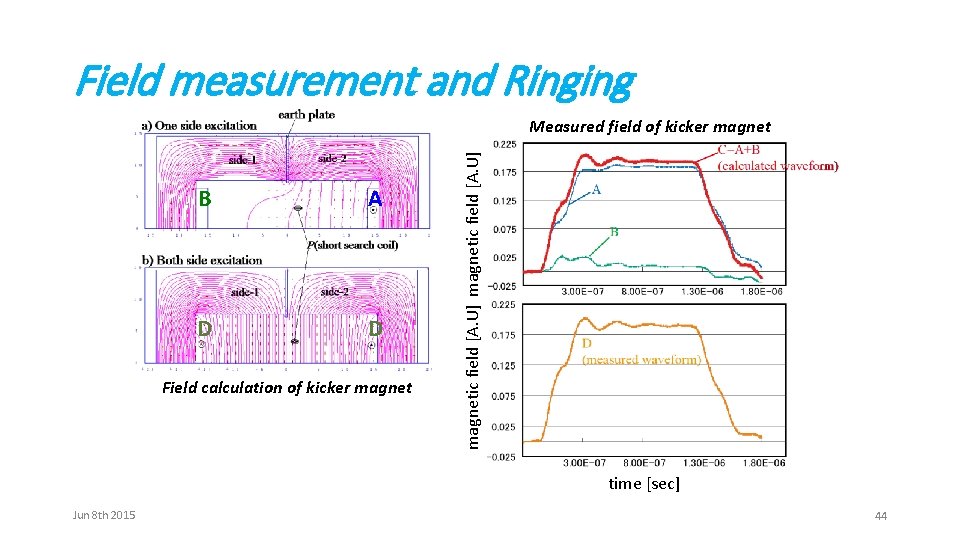
Field measurement and Ringing B A D D Field calculation of kicker magnetic field [A. U] Measured field of kicker magnet time [sec] Jun 8 th 2015 44
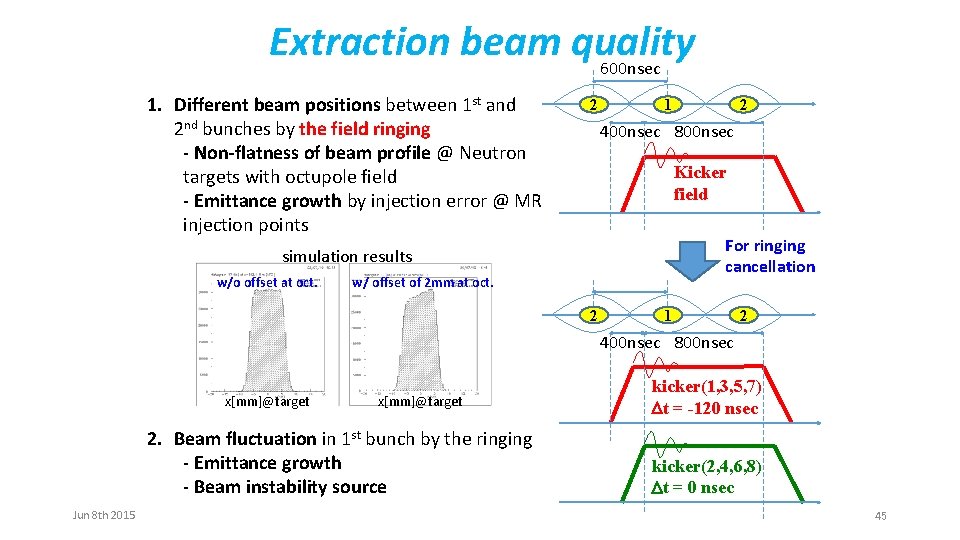
Extraction beam quality 600 nsec 1. Different beam positions between 1 st and 2 nd bunches by the field ringing - Non-flatness of beam profile @ Neutron targets with octupole field - Emittance growth by injection error @ MR injection points 2 1 400 nsec 800 nsec Kicker field For ringing cancellation simulation results w/o offset at oct. 2 w/ offset of 2 mm at oct. 2 1 2 400 nsec 800 nsec x[mm]@target 2. Beam fluctuation in 1 st bunch by the ringing - Emittance growth - Beam instability source Jun 8 th 2015 kicker(1, 3, 5, 7) Dt = -120 nsec kicker(2, 4, 6, 8) Dt = 0 nsec 45
![Beam displacement measurement caused by kicker field ringing Measured Dxmm Beam condition shorter Beam displacement measurement caused by kicker field ringing Measured Dx[mm] Beam condition : shorter](https://slidetodoc.com/presentation_image_h2/5d0aad23d25bb3e3627177fa406188e3/image-46.jpg)
Beam displacement measurement caused by kicker field ringing Measured Dx[mm] Beam condition : shorter single bunched beam (~150 nsec→~30 nsec) Monitor : Beam Position Monitor (BPM) @ extraction beam transport line Knob : Fire timing (Dt) of all kickers Kicker field +3. 3 mm -5. 5 mm Dt [nsec] Beam center positions of both 1 st and 2 nd bunches are ~0 mm. Beam fluctuation of 1 st bunch is from -5. 5 (-1. 6%) to +3. 3 mm(+0. 75%). Jun 8 th 2015 Time structure of the ringing is not simple! 46
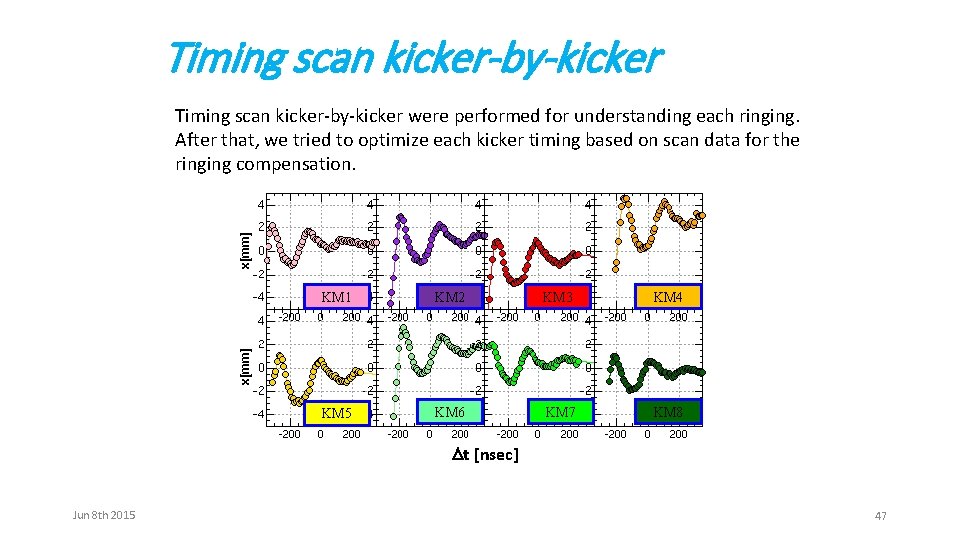
Timing scan kicker-by-kicker were performed for understanding each ringing. After that, we tried to optimize each kicker timing based on scan data for the ringing compensation. KM 1 KM 2 KM 3 KM 4 KM 5 KM 6 KM 7 KM 8 Dt [nsec] Jun 8 th 2015 47
![ID ① ΔT ② ΔT ① KM 1 0 nsec 10 nsec Measured Dxmm ID ① ΔT ② ΔT ① KM 1 0 nsec 10 nsec Measured Dx[mm]](https://slidetodoc.com/presentation_image_h2/5d0aad23d25bb3e3627177fa406188e3/image-48.jpg)
ID ① ΔT ② ΔT ① KM 1 0 nsec 10 nsec Measured Dx[mm] Comparison between all and each kicker timing scan KM 2 0 nsec KM 3 0 nsec KM 4 0 nsec 35 nsec KM 5 0 nsec KM 6 0 nsec KM 7 0 nsec 10 nsec KM 8 0 nsec -10 nsec : Summed positions for each scan : Measured position for all scan Measured Dx[mm] ② Jun 8 th 2015 Summed scan position for each KMs ( ) is a good agreement with measured position for all KM timing ( ). ) ⇒ We can discuss with kicker ringing compensation by using each scan data. timing ΔT [nsec] 48
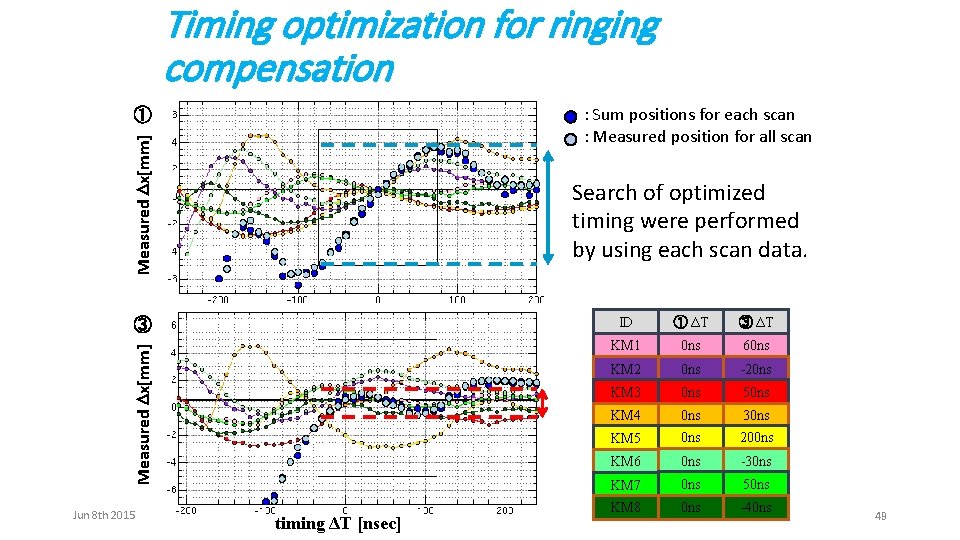
Timing optimization for ringing compensation : Sum positions for each scan : Measured position for all scan Measured Dx[mm] ① ③ ID ① ΔT ③ ΔT Measured Dx[mm] Search of optimized timing were performed by using each scan data. KM 1 0 ns 60 ns KM 2 0 ns -20 ns KM 3 0 ns 50 ns KM 4 0 ns 30 ns KM 5 0 ns 200 ns KM 6 0 ns -30 ns KM 7 0 ns 50 ns KM 8 0 ns -40 ns Jun 8 th 2015 timing ΔT [nsec] 49
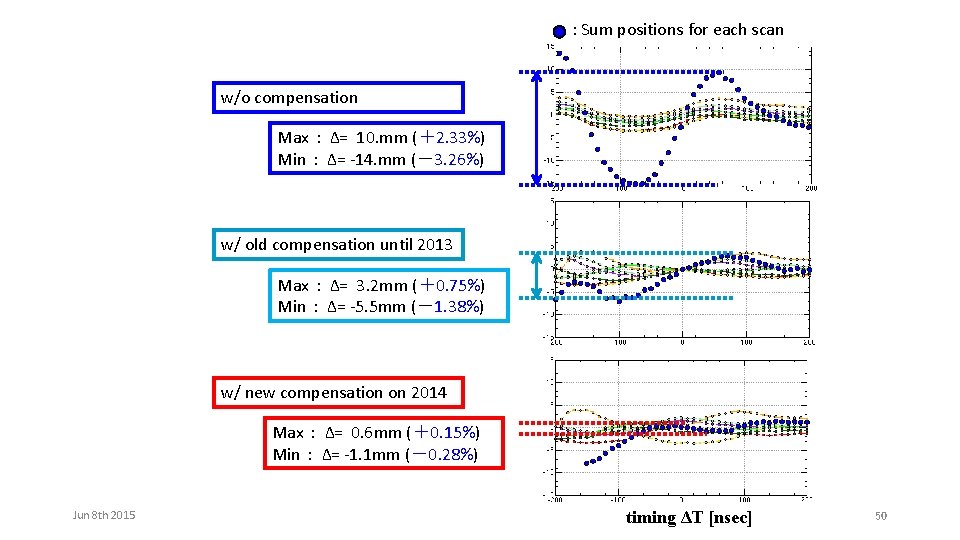
: Sum positions for each scan w/o compensation Max : Δ= 10. mm (+2. 33%) Min : Δ= -14. mm (-3. 26%) w/ old compensation until 2013 Max : Δ= 3. 2 mm (+0. 75%) Min : Δ= -5. 5 mm (-1. 38%) w/ new compensation on 2014 Max : Δ= 0. 6 mm (+0. 15%) Min : Δ= -1. 1 mm (-0. 28%) Jun 8 th 2015 timing ΔT [nsec] 50
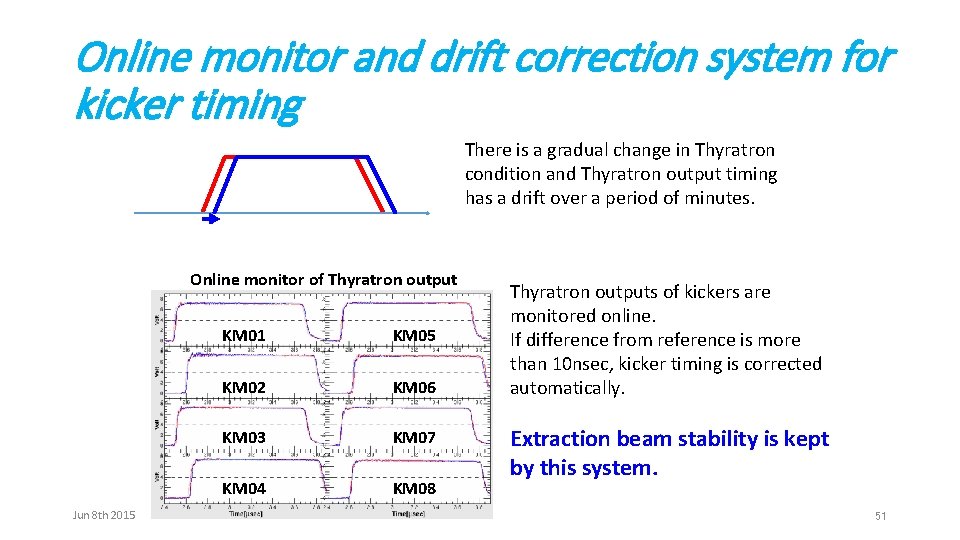
Online monitor and drift correction system for kicker timing There is a gradual change in Thyratron condition and Thyratron output timing has a drift over a period of minutes. Online monitor of Thyratron output Jun 8 th 2015 KM 01 KM 05 KM 02 KM 06 KM 03 KM 07 KM 04 KM 08 Thyratron outputs of kickers are monitored online. If difference from reference is more than 10 nsec, kicker timing is corrected automatically. Extraction beam stability is kept by this system. 51
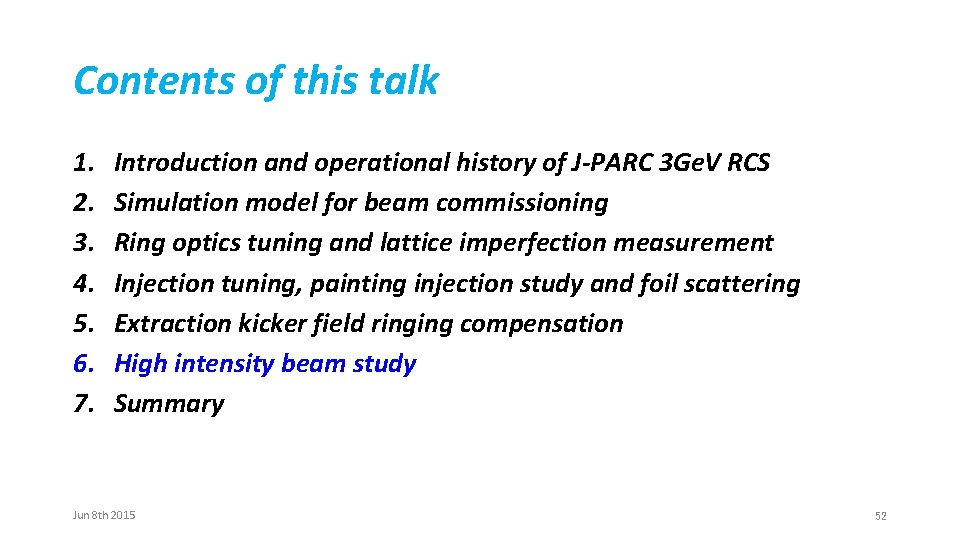
Contents of this talk 1. 2. 3. 4. 5. 6. 7. Introduction and operational history of J-PARC 3 Ge. V RCS Simulation model for beam commissioning Ring optics tuning and lattice imperfection measurement Injection tuning, painting injection study and foil scattering Extraction kicker field ringing compensation High intensity beam study Summary Jun 8 th 2015 52
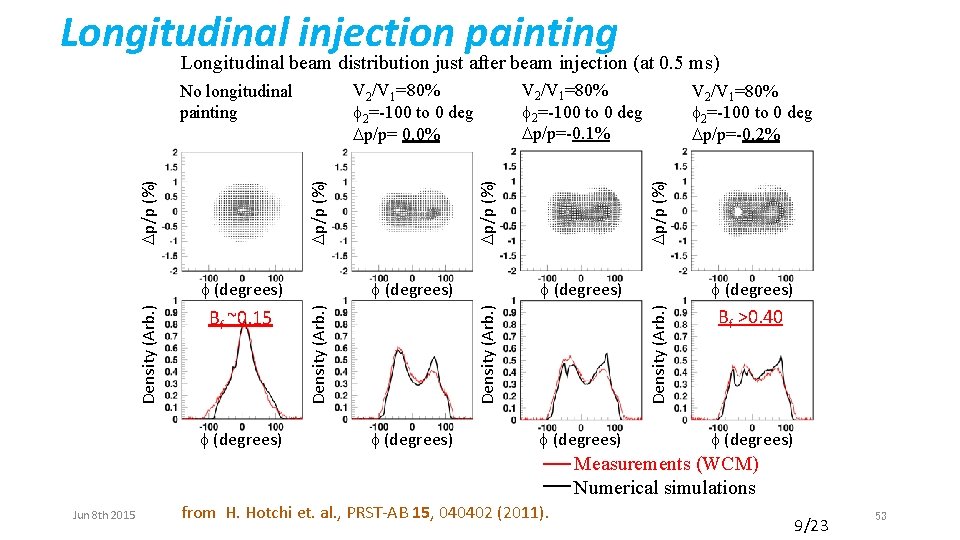
Longitudinal injection painting Longitudinal beam distribution just after beam injection (at 0. 5 ms) f (degrees) Density (Arb. ) f (degrees) V 2/V 1=80% f 2=-100 to 0 deg Dp/p=-0. 2% Dp/p (%) Density (Arb. ) f (degrees) Bf ~0. 15 V 2/V 1=80% f 2=-100 to 0 deg Dp/p=-0. 1% V 2/V 1=80% f 2=-100 to 0 deg Dp/p= 0. 0% No longitudinal painting f (degrees) Bf >0. 40 f (degrees) Measurements (WCM) Numerical simulations Jun 8 th 2015 from H. Hotchi et. al. , PRST-AB 15, 040402 (2011). 9/23 53
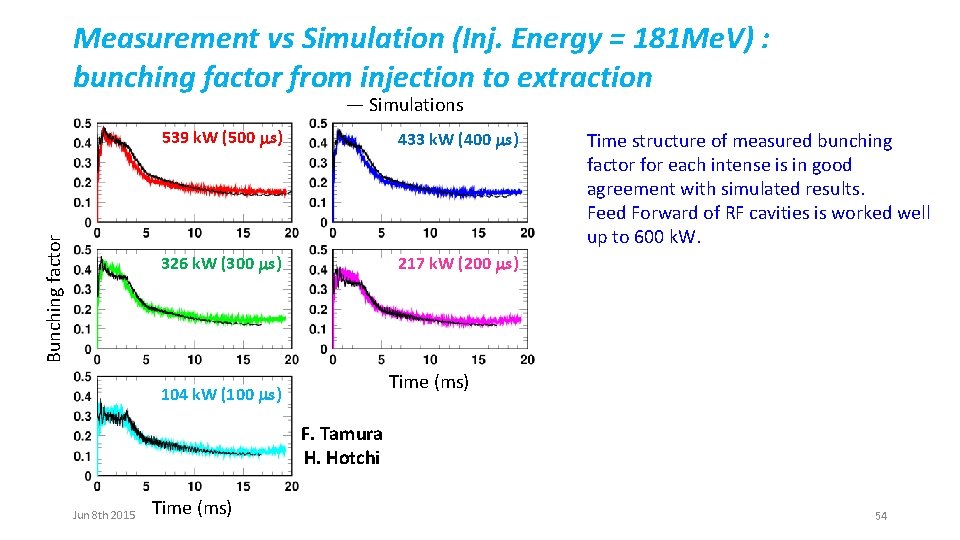
Measurement vs Simulation (Inj. Energy = 181 Me. V) : bunching factor from injection to extraction Bunching factor ― Simulations 539 k. W (500 ms) 433 k. W (400 ms) 326 k. W (300 ms) 217 k. W (200 ms) Time structure of measured bunching factor for each intense is in good agreement with simulated results. Feed Forward of RF cavities is worked well up to 600 k. W. Time (ms) 104 k. W (100 ms) F. Tamura H. Hotchi Jun 8 th 2015 Time (ms) 54
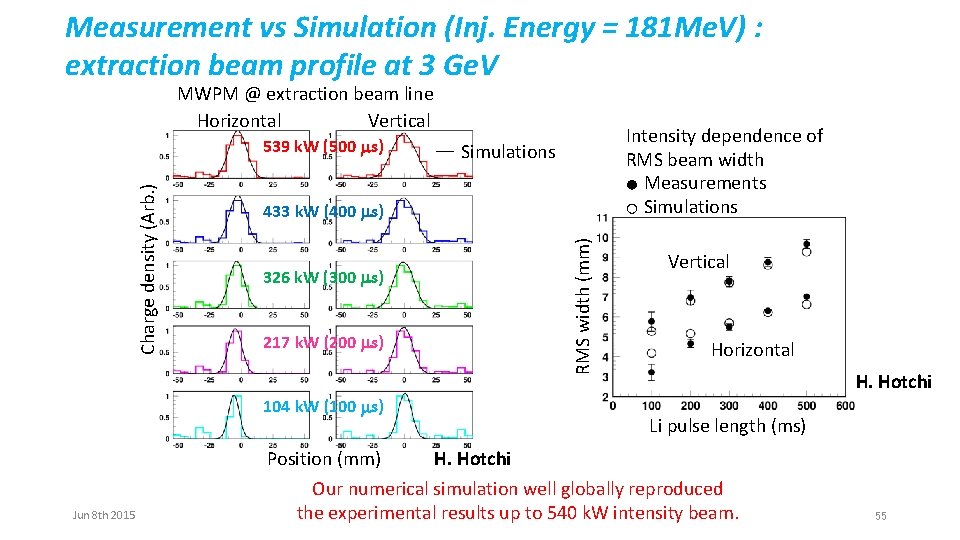
Measurement vs Simulation (Inj. Energy = 181 Me. V) : extraction beam profile at 3 Ge. V MWPM @ extraction beam line Vertical Horizontal ― Simulations 433 k. W (400 ms) 326 k. W (300 ms) 217 k. W (200 ms) 104 k. W (100 ms) Jun 8 th 2015 Intensity dependence of RMS beam width ● Measurements ○ Simulations RMS width (mm) Charge density (Arb. ) 539 k. W (500 ms) Vertical Horizontal H. Hotchi Li pulse length (ms) Position (mm) H. Hotchi Our numerical simulation well globally reproduced the experimental results up to 540 k. W intensity beam. 55
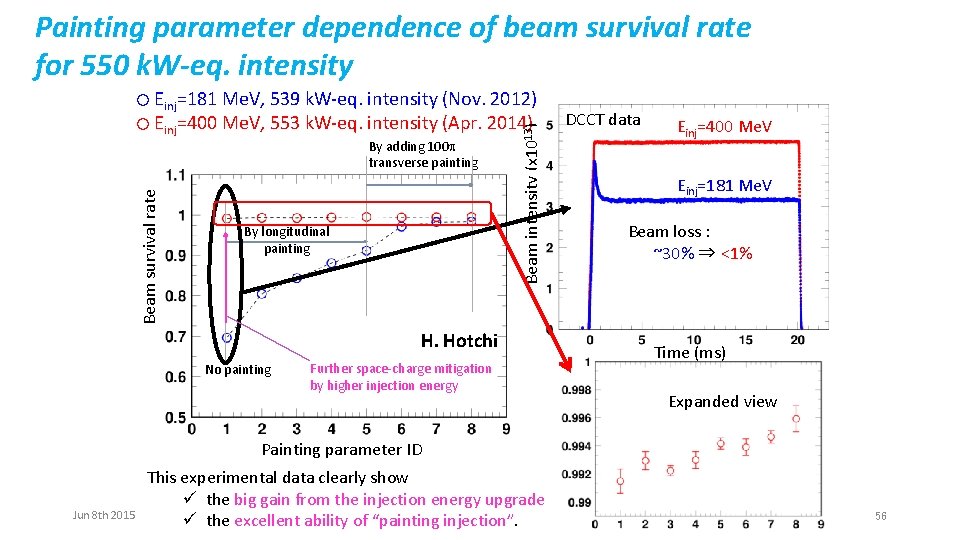
Painting parameter dependence of beam survival rate for 550 k. W-eq. intensity Einj=181 Me. V, 539 k. W-eq. intensity (Nov. 2012) ○ Einj=400 Me. V, 553 k. W-eq. intensity (Apr. 2014) Beam survival rate By adding 100 p transverse painting By longitudinal painting Beam intensity (x 1013) ○ H. Hotchi No painting Further space-charge mitigation by higher injection energy DCCT data Einj=400 Me. V Einj=181 Me. V Beam loss : ~30% ⇒ <1% Time (ms) Expanded view Painting parameter ID Jun 8 th 2015 This experimental data clearly show ü the big gain from the injection energy upgrade ü the excellent ability of “painting injection”. 56
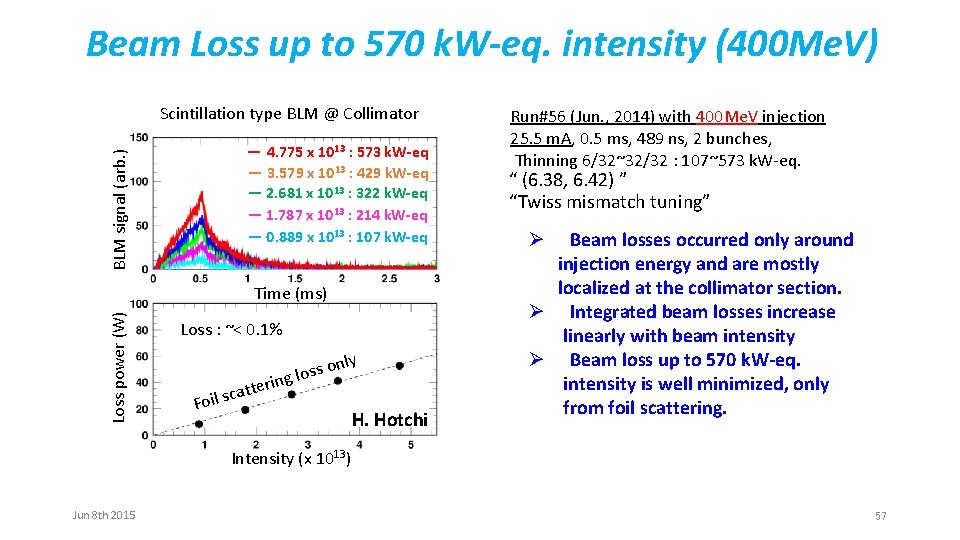
Beam Loss up to 570 k. W-eq. intensity (400 Me. V) BLM signal (arb. ) Scintillation type BLM @ Collimator ― 4. 775 x 1013 : 573 k. W-eq ― 3. 579 x 1013 : 429 k. W-eq ― 2. 681 x 1013 : 322 k. W-eq ― 1. 787 x 1013 : 214 k. W-eq ― 0. 889 x 1013 : 107 k. W-eq Loss power (W) Time (ms) Loss : ~< 0. 1% ly s on s o l ing ter t a c s Foil H. Hotchi Run#56 (Jun. , 2014) with 400 Me. V injection 25. 5 m. A, 0. 5 ms, 489 ns, 2 bunches, Thinning 6/32~32/32 : 107~573 k. W-eq. “ (6. 38, 6. 42) ” “Twiss mismatch tuning” Beam losses occurred only around injection energy and are mostly localized at the collimator section. Ø Integrated beam losses increase linearly with beam intensity Ø Beam loss up to 570 k. W-eq. intensity is well minimized, only from foil scattering. Ø Intensity (x 1013) Jun 8 th 2015 57
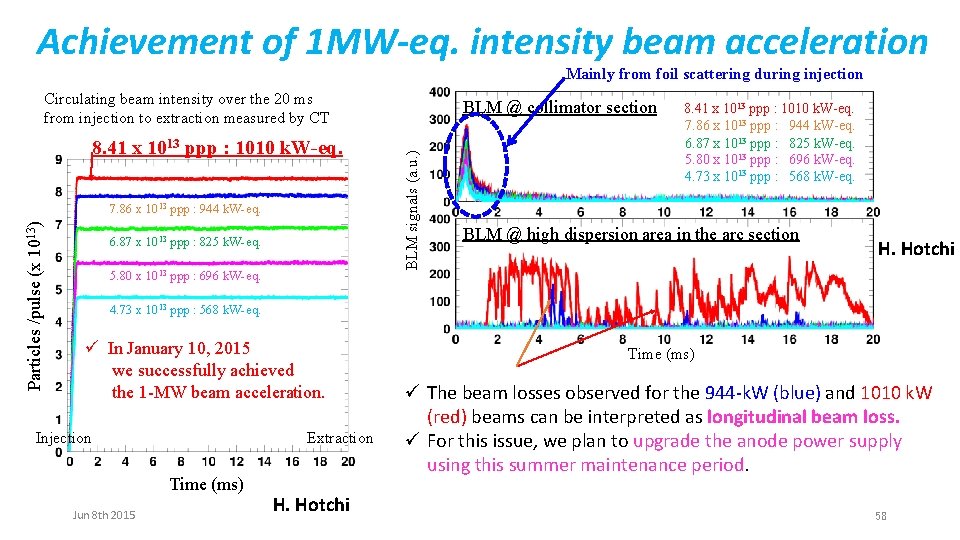
Achievement of 1 MW-eq. intensity beam acceleration Mainly from foil scattering during injection Circulating beam intensity over the 20 ms from injection to extraction measured by CT Particles /pulse (x 1013) 7. 86 x 1013 ppp : 944 k. W-eq. 6. 87 x 1013 ppp : 825 k. W-eq. 5. 80 x 1013 ppp : 696 k. W-eq. BLM signals (a. u. ) 8. 41 x 1013 ppp : 1010 k. W-eq. BLM @ collimator section 8. 41 x 1013 ppp : 1010 k. W-eq. 7. 86 x 1013 ppp : 944 k. W-eq. 6. 87 x 1013 ppp : 825 k. W-eq. 5. 80 x 1013 ppp : 696 k. W-eq. 4. 73 x 1013 ppp : 568 k. W-eq. BLM @ high dispersion area in the arc section H. Hotchi 4. 73 x 1013 ppp : 568 k. W-eq. ü In January 10, 2015 we successfully achieved the 1 -MW beam acceleration. Injection Extraction Time (ms) Jun 8 th 2015 H. Hotchi Time (ms) ü The beam losses observed for the 944 -k. W (blue) and 1010 k. W (red) beams can be interpreted as longitudinal beam loss. ü For this issue, we plan to upgrade the anode power supply using this summer maintenance period. 58
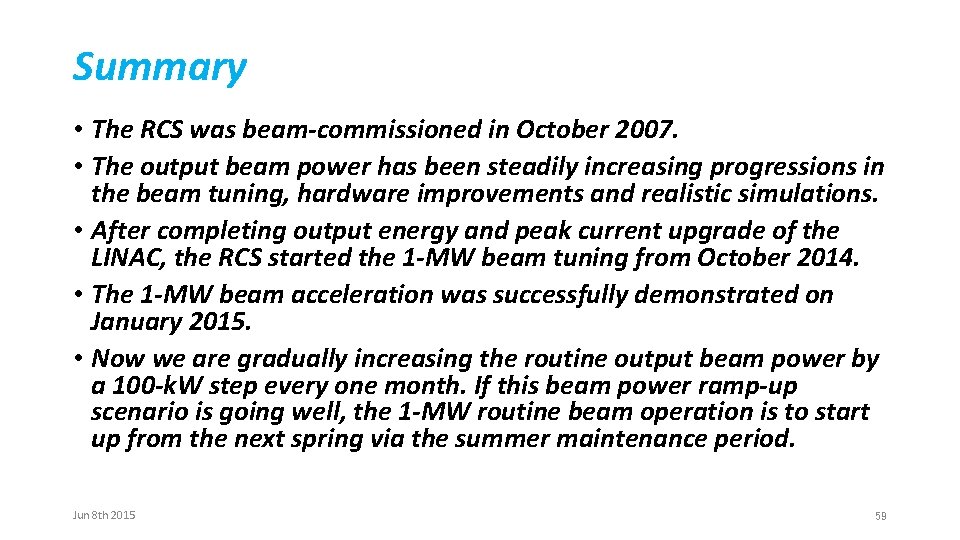
Summary • The RCS was beam-commissioned in October 2007. • The output beam power has been steadily increasing progressions in the beam tuning, hardware improvements and realistic simulations. • After completing output energy and peak current upgrade of the LINAC, the RCS started the 1 -MW beam tuning from October 2014. • The 1 -MW beam acceleration was successfully demonstrated on January 2015. • Now we are gradually increasing the routine output beam power by a 100 -k. W step every one month. If this beam power ramp-up scenario is going well, the 1 -MW routine beam operation is to start up from the next spring via the summer maintenance period. Jun 8 th 2015 59
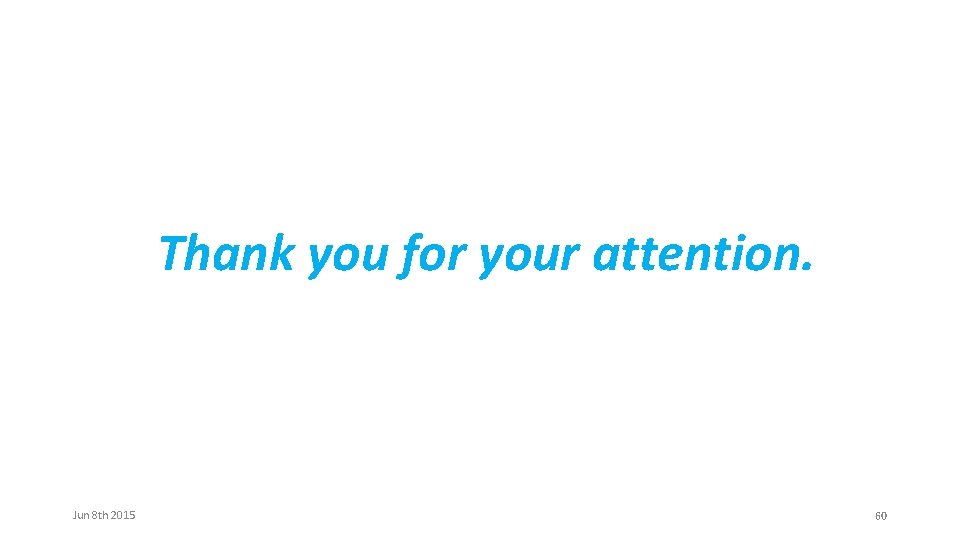
Thank you for your attention. Jun 8 th 2015 60
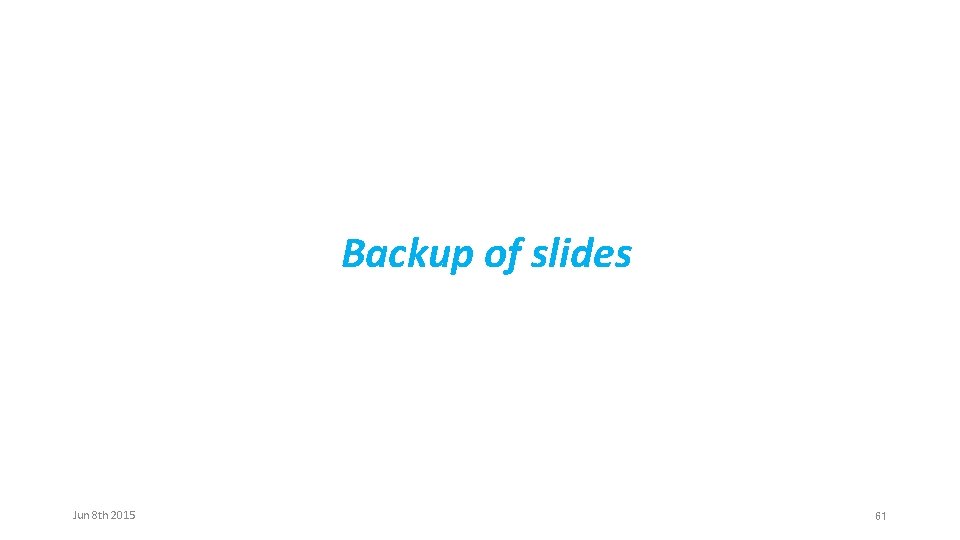
Backup of slides Jun 8 th 2015 61
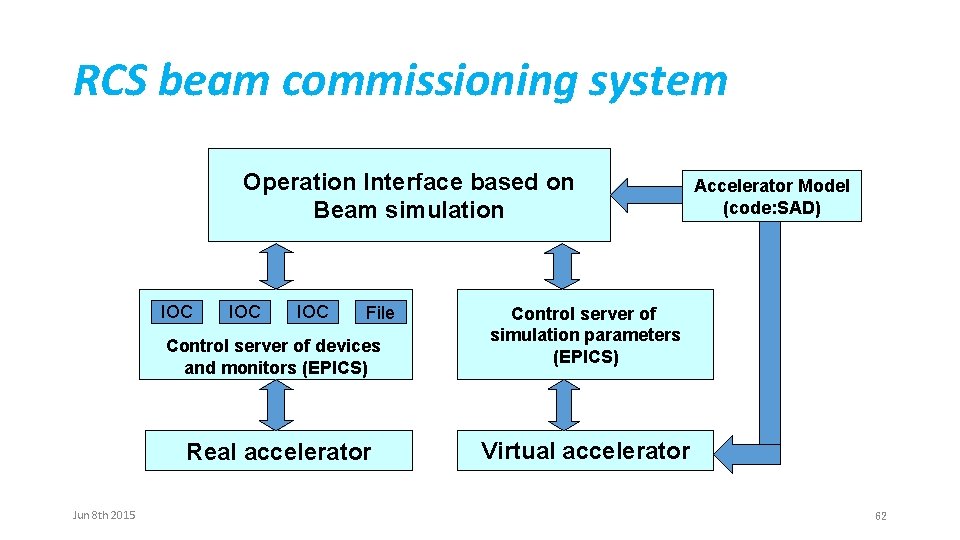
RCS beam commissioning system Operation Interface based on Operation Interface Beam simulation IOC IOC File Control server of devices and monitors (EPICS) Real accelerator Jun 8 th 2015 Accelerator Model (code: SAD) Control server of simulation parameters (EPICS) Virtual accelerator 62
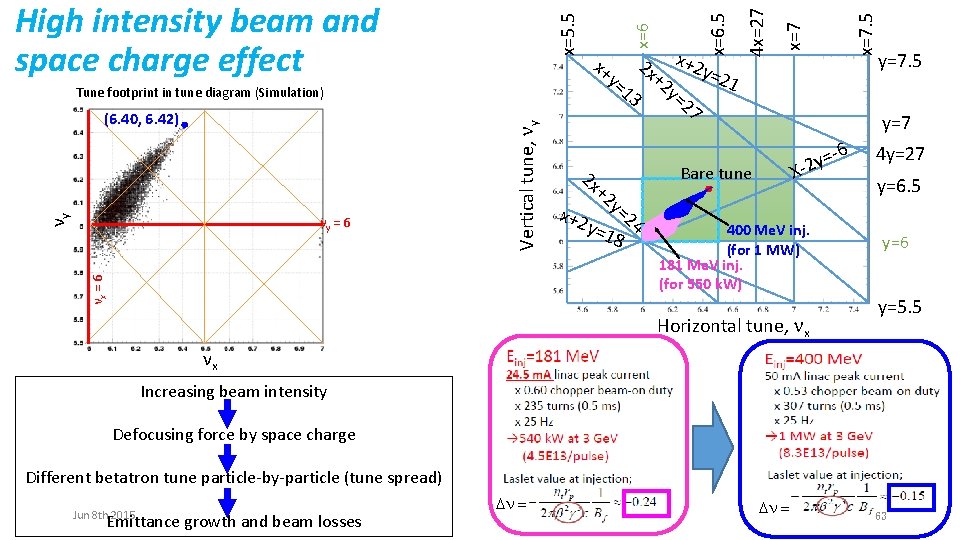
y= νy (6. 40, 6. 42) νx = 6 νy = 6 Vertical tune, ny Tune footprint in tune diagram (Simulation) 2 x +2 x+2 13 y= 2 y=1 4 8 +2 y= 27 y=2 x=7. 5 x+2 x=7 2 x 4 x=27 x+ x=6. 5 x=6 x=5. 5 High intensity beam and space charge effect 1 y=7. 5 y=7 = y X-2 Bare tune 400 Me. V inj. (for 1 MW) 181 Me. V inj. (for 550 k. W) Horizontal tune, nx 6 4 y=27 y=6. 5 y=6 y=5. 5 νx Increasing beam intensity Defocusing force by space charge Different betatron tune particle-by-particle (tune spread) Jun 8 th 2015 Emittance growth and beam losses Dn = 63
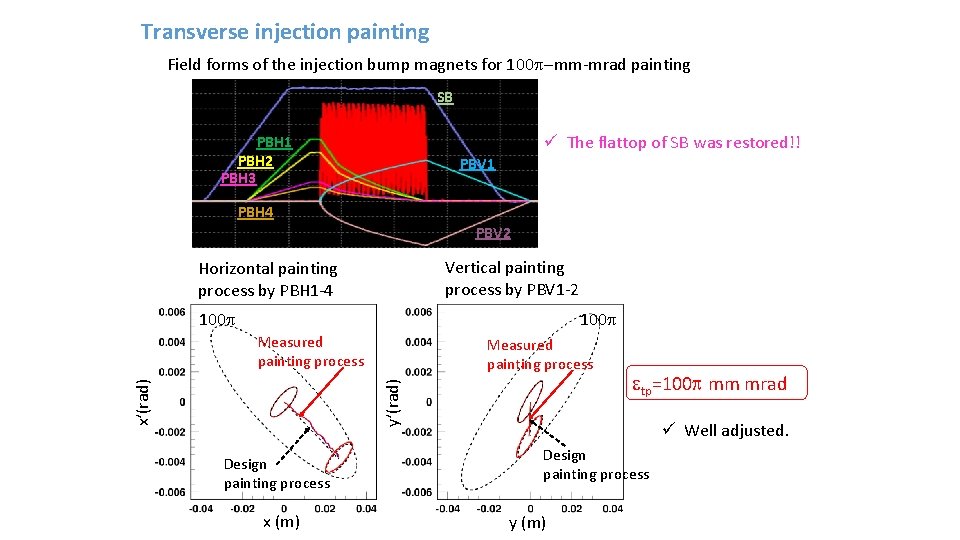
Transverse injection painting Field forms of the injection bump magnets for 100 p-mm-mrad painting SB ü The flattop of SB was restored!! PBH 1 PBH 2 PBH 3 PBV 1 PBH 4 PBV 2 Vertical painting process by PBV 1 -2 Horizontal painting process by PBH 1 -4 100 p Measured painting process x’(rad) y’(rad) Measured painting process Design painting process x (m) etp=100 p mm mrad ü Well adjusted. Design painting process y (m)
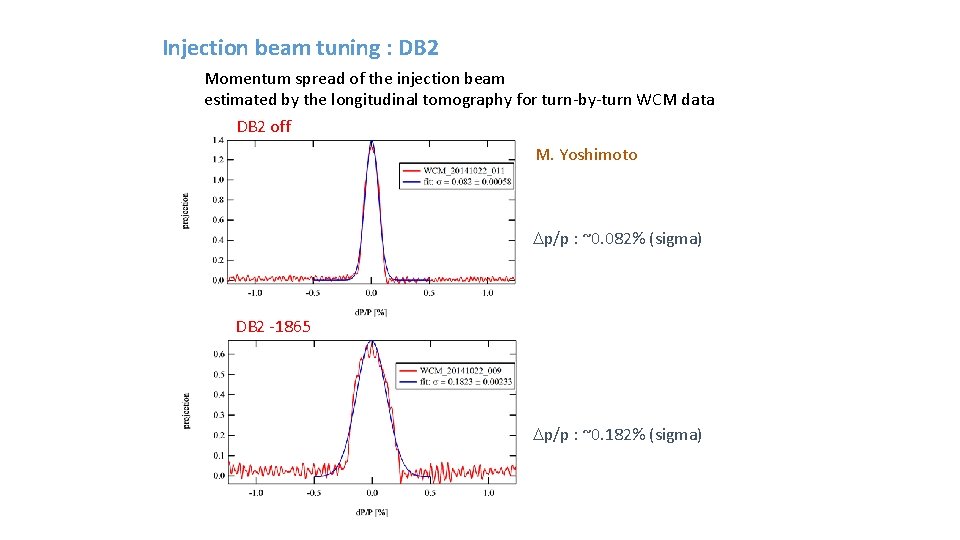
Injection beam tuning : DB 2 Momentum spread of the injection beam estimated by the longitudinal tomography for turn-by-turn WCM data DB 2 off M. Yoshimoto Dp/p : ~0. 082% (sigma) DB 2 -1865 Dp/p : ~0. 182% (sigma)
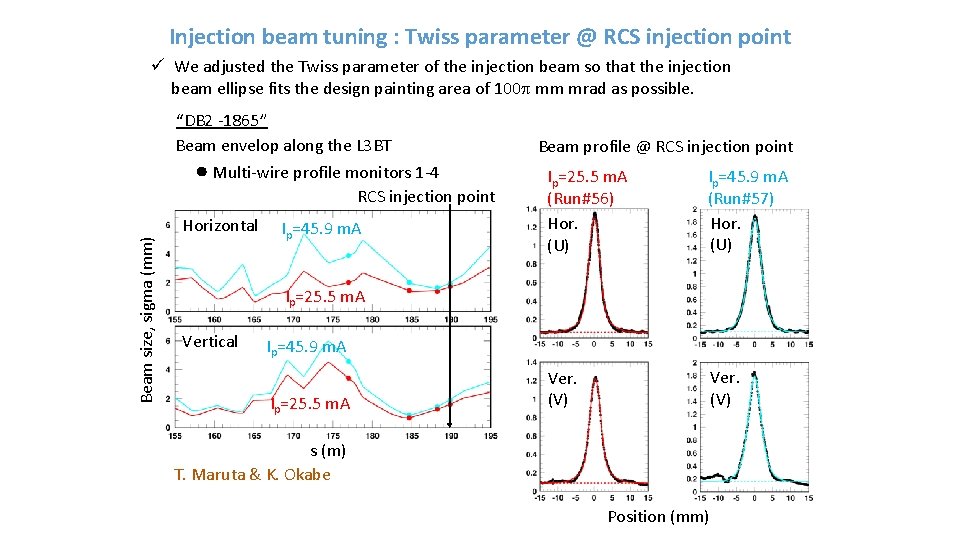
Injection beam tuning : Twiss parameter @ RCS injection point ü We adjusted the Twiss parameter of the injection beam so that the injection beam ellipse fits the design painting area of 100 p mm mrad as possible. “DB 2 -1865” Beam envelop along the L 3 BT Multi-wire profile monitors 1 -4 RCS injection point Beam size, sigma (mm) Horizontal Ip=45. 9 m. A Beam profile @ RCS injection point Ip=25. 5 m. A (Run#56) Hor. (U) Ip=45. 9 m. A (Run#57) Hor. (U) Ver. (V) Ip=25. 5 m. A Vertical Ip=45. 9 m. A Ip=25. 5 m. A s (m) T. Maruta & K. Okabe Position (mm)
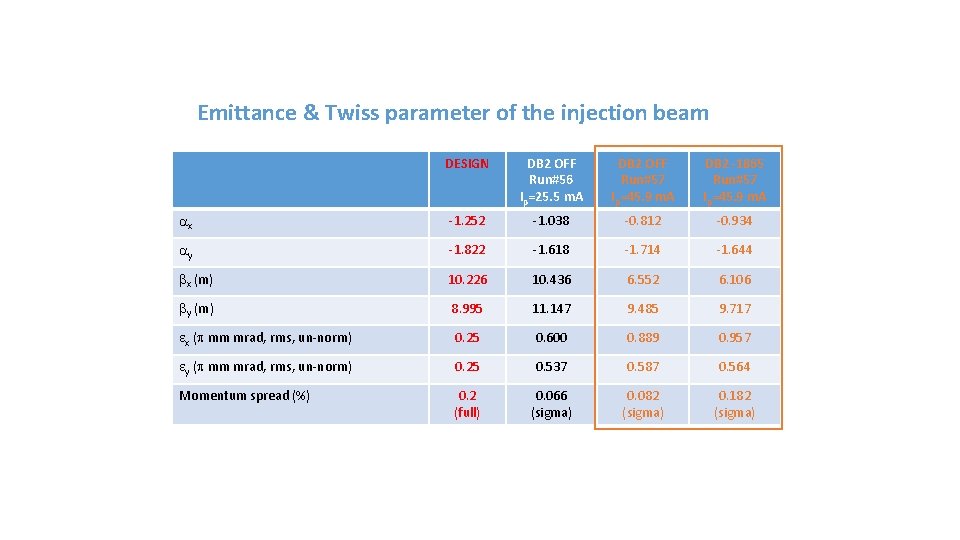
Emittance & Twiss parameter of the injection beam DESIGN DB 2 OFF Run#56 Ip=25. 5 m. A DB 2 OFF Run#57 Ip=45. 9 m. A DB 2 -1865 Run#57 Ip=45. 9 m. A ax -1. 252 -1. 038 -0. 812 -0. 934 ay -1. 822 -1. 618 -1. 714 -1. 644 bx (m) 10. 226 10. 436 6. 552 6. 106 by (m) 8. 995 11. 147 9. 485 9. 717 ex (p mm mrad, rms, un-norm) 0. 25 0. 600 0. 889 0. 957 ey (p mm mrad, rms, un-norm) 0. 25 0. 537 0. 587 0. 564 Momentum spread (%) 0. 2 (full) 0. 066 (sigma) 0. 082 (sigma) 0. 182 (sigma)
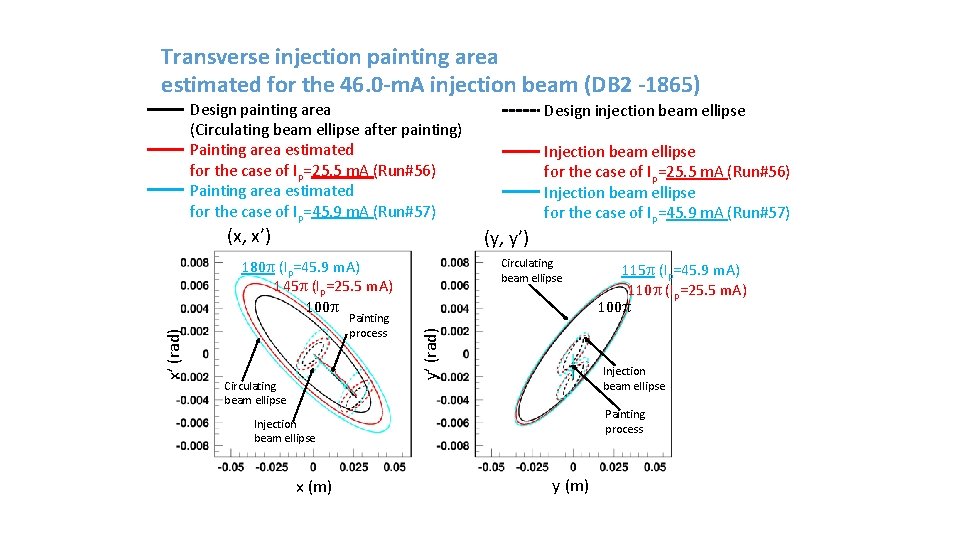
Transverse injection painting area estimated for the 46. 0 -m. A injection beam (DB 2 -1865) Design painting area (Circulating beam ellipse after painting) Painting area estimated for the case of Ip=25. 5 m. A (Run#56) Painting area estimated for the case of Ip=45. 9 m. A (Run#57) (x, x’) (y, y’) Circulating beam ellipse y’ (rad) Painting process Injection beam ellipse for the case of Ip=25. 5 m. A (Run#56) Injection beam ellipse for the case of Ip=45. 9 m. A (Run#57) Circulating beam ellipse 180 p (Ip=45. 9 m. A) 145 p (Ip=25. 5 m. A) 100 p x’ (rad) Design injection beam ellipse Injection beam ellipse Painting process Injection beam ellipse x (m) 115 p (Ip=45. 9 m. A) 110 p (Ip=25. 5 m. A) 100 p y (m)
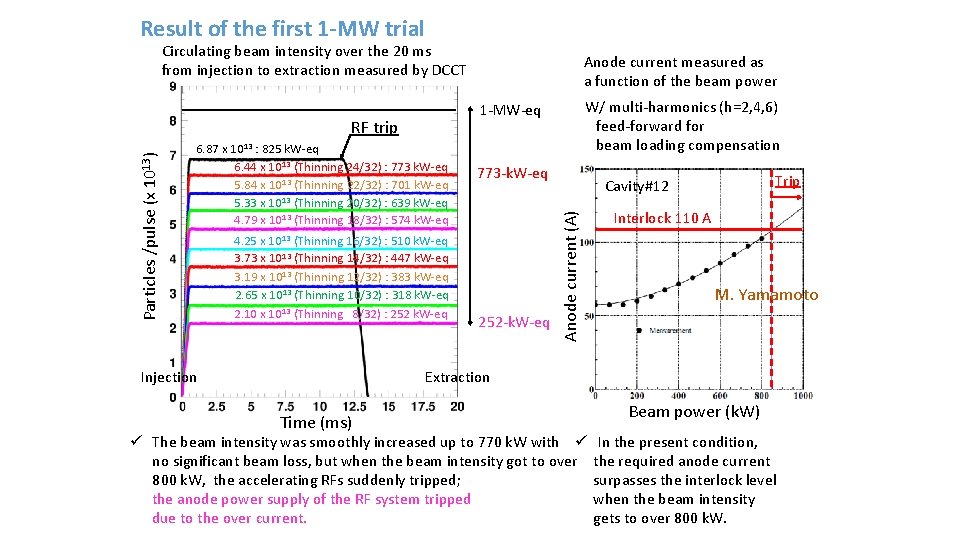
Result of the first 1 -MW trial Circulating beam intensity over the 20 ms from injection to extraction measured by DCCT 6. 87 x 1013 : 825 k. W-eq 6. 44 x 1013 (Thinning 24/32) : 773 k. W-eq 5. 84 x 1013 (Thinning 22/32) : 701 k. W-eq 5. 33 x 1013 (Thinning 20/32) : 639 k. W-eq 4. 79 x 1013 (Thinning 18/32) : 574 k. W-eq 4. 25 x 1013 (Thinning 16/32) : 510 k. W-eq 3. 73 x 1013 (Thinning 14/32) : 447 k. W-eq 3. 19 x 1013 (Thinning 12/32) : 383 k. W-eq 2. 65 x 1013 (Thinning 10/32) : 318 k. W-eq 2. 10 x 1013 (Thinning 8/32) : 252 k. W-eq 773 -k. W-eq 252 -k. W-eq Trip Cavity#12 Anode current (A) Particles /pulse (x 1013) W/ multi-harmonics (h=2, 4, 6) feed-forward for beam loading compensation 1 -MW-eq RF trip Injection Anode current measured as a function of the beam power Interlock 110 A M. Yamamoto Extraction Time (ms) ü The beam intensity was smoothly increased up to 770 k. W with ü no significant beam loss, but when the beam intensity got to over 800 k. W, the accelerating RFs suddenly tripped; the anode power supply of the RF system tripped due to the over current. Beam power (k. W) In the present condition, the required anode current surpasses the interlock level when the beam intensity gets to over 800 k. W.
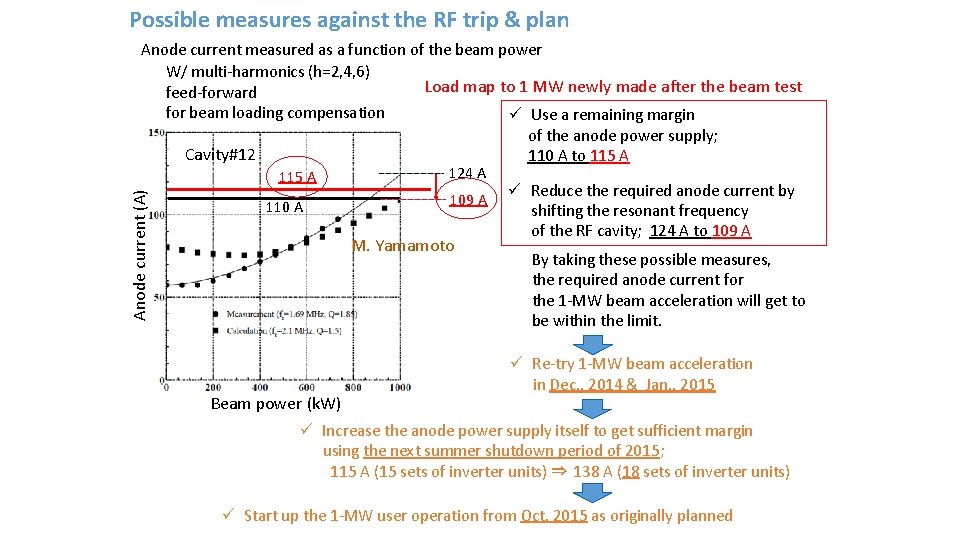
Possible measures against the RF trip & plan Anode current measured as a function of the beam power W/ multi-harmonics (h=2, 4, 6) Load map to 1 MW newly made after the beam test feed-forward for beam loading compensation ü Use a remaining margin of the anode power supply; Cavity#12 110 A to 115 A Anode current (A) 115 A 110 A 124 A 109 A M. Yamamoto Beam power (k. W) ü Reduce the required anode current by shifting the resonant frequency of the RF cavity; 124 A to 109 A By taking these possible measures, the required anode current for the 1 -MW beam acceleration will get to be within the limit. ü Re-try 1 -MW beam acceleration in Dec. , 2014 & Jan. , 2015 ü Increase the anode power supply itself to get sufficient margin using the next summer shutdown period of 2015; 115 A (15 sets of inverter units) ⇒ 138 A (18 sets of inverter units) ü Start up the 1 -MW user operation from Oct. 2015 as originally planned
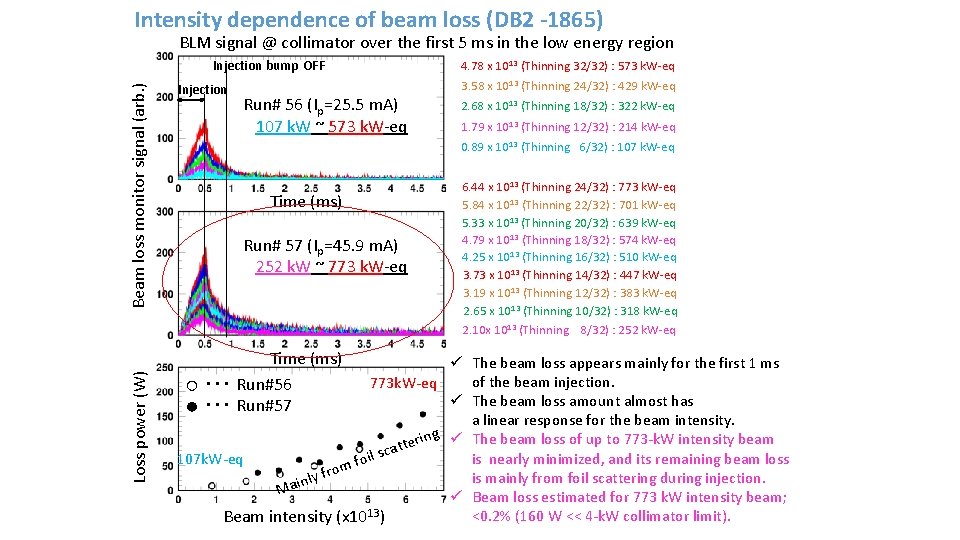
Intensity dependence of beam loss (DB 2 -1865) BLM signal @ collimator over the first 5 ms in the low energy region Loss power (W) Beam loss monitor signal (arb. ) Injection bump OFF Injection Run# 56 (Ip=25. 5 m. A) 107 k. W ~ 573 k. W-eq 4. 78 x 1013 (Thinning 32/32) : 573 k. W-eq 3. 58 x 1013 (Thinning 24/32) : 429 k. W-eq 2. 68 x 1013 (Thinning 18/32) : 322 k. W-eq 1. 79 x 1013 (Thinning 12/32) : 214 k. W-eq 0. 89 x 1013 (Thinning 6/32) : 107 k. W-eq Time (ms) Run# 57 (Ip=45. 9 m. A) 252 k. W ~ 773 k. W-eq Time (ms) ・・・ Run#56 ・・・ Run#57 6. 44 x 1013 (Thinning 24/32) : 773 k. W-eq 5. 84 x 1013 (Thinning 22/32) : 701 k. W-eq 5. 33 x 1013 (Thinning 20/32) : 639 k. W-eq 4. 79 x 1013 (Thinning 18/32) : 574 k. W-eq 4. 25 x 1013 (Thinning 16/32) : 510 k. W-eq 3. 73 x 1013 (Thinning 14/32) : 447 k. W-eq 3. 19 x 1013 (Thinning 12/32) : 383 k. W-eq 2. 65 x 1013 (Thinning 10/32) : 318 k. W-eq 2. 10 x 1013 (Thinning 8/32) : 252 k. W-eq ü The beam loss appears mainly for the first 1 ms of the beam injection. 773 k. W-eq ○ ü The beam loss amount almost has ● a linear response for the beam intensity. g erin ü The beam loss of up to 773 -k. W intensity beam t t a il sc is nearly minimized, and its remaining beam loss 107 k. W-eq o f m o r is mainly from foil scattering during injection. nly f i a M ü Beam loss estimated for 773 k. W intensity beam; <0. 2% (160 W << 4 -k. W collimator limit). Beam intensity (x 1013)
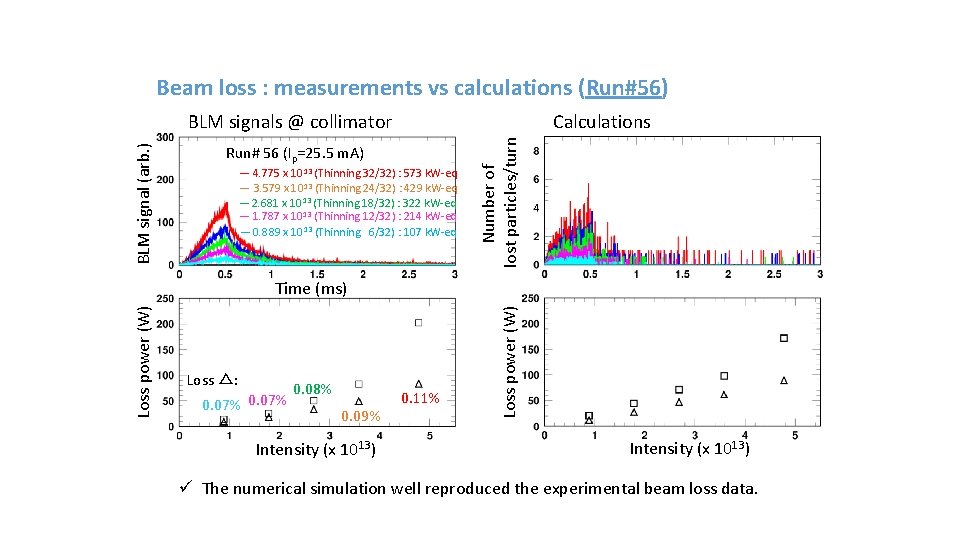
Beam loss : measurements vs calculations (Run#56) Calculations Run# 56 (Ip=25. 5 m. A) ― 4. 775 x 10 13 (Thinning 32/32) : 573 k. W-eq ― 3. 579 x 10 13 (Thinning 24/32) : 429 k. W-eq ― 2. 681 x 10 13 (Thinning 18/32) : 322 k. W-eq ― 1. 787 x 10 13 (Thinning 12/32) : 214 k. W-eq ― 0. 889 x 10 13 (Thinning 6/32) : 107 k. W-eq Number of lost particles/turn BLM signal (arb. ) BLM signals @ collimator Loss △: 0. 07% 0. 08% 0. 09% Intensity (x 1013) 0. 11% Loss power (W) Time (ms) Intensity (x 1013) ü The numerical simulation well reproduced the experimental beam loss data.
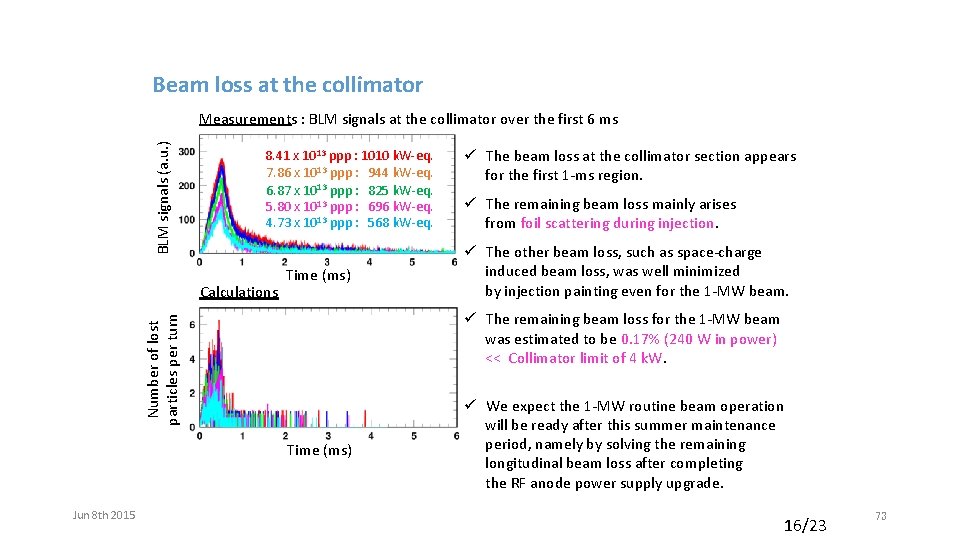
Beam loss at the collimator BLM signals (a. u. ) Measurements : BLM signals at the collimator over the first 6 ms 8. 41 x 1013 ppp : 1010 k. W-eq. 7. 86 x 1013 ppp : 944 k. W-eq. 6. 87 x 1013 ppp : 825 k. W-eq. 5. 80 x 1013 ppp : 696 k. W-eq. 4. 73 x 1013 ppp : 568 k. W-eq. Time (ms) Jun 8 th 2015 ü The remaining beam loss mainly arises from foil scattering during injection. ü The other beam loss, such as space-charge induced beam loss, was well minimized by injection painting even for the 1 -MW beam. ü The remaining beam loss for the 1 -MW beam was estimated to be 0. 17% (240 W in power) << Collimator limit of 4 k. W. Number of lost particles per turn Calculations Time (ms) ü The beam loss at the collimator section appears for the first 1 -ms region. ü We expect the 1 -MW routine beam operation will be ready after this summer maintenance period, namely by solving the remaining longitudinal beam loss after completing the RF anode power supply upgrade. 16/23 73
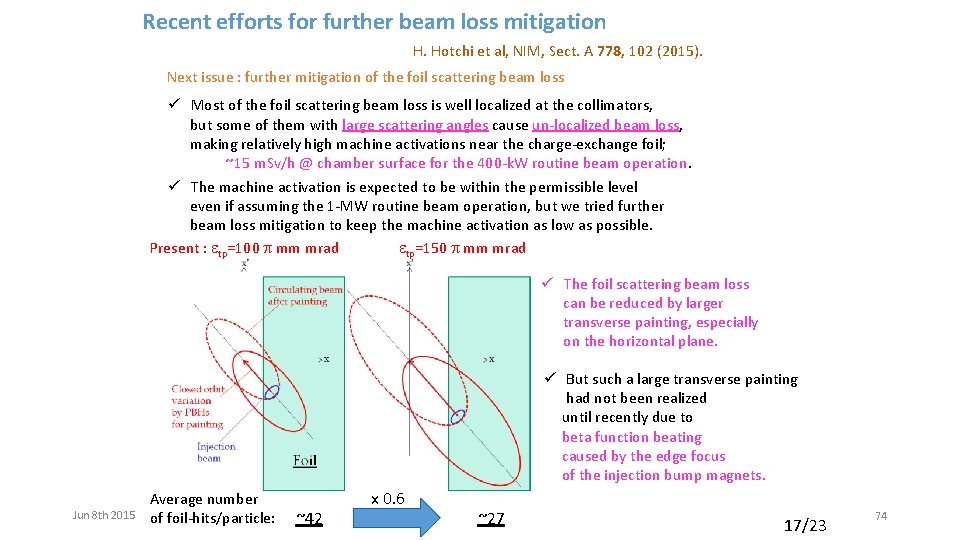
Recent efforts for further beam loss mitigation H. Hotchi et al, NIM, Sect. A 778, 102 (2015). Next issue : further mitigation of the foil scattering beam loss ü Most of the foil scattering beam loss is well localized at the collimators, but some of them with large scattering angles cause un-localized beam loss, making relatively high machine activations near the charge-exchange foil; ~15 m. Sv/h @ chamber surface for the 400 -k. W routine beam operation. ü The machine activation is expected to be within the permissible level even if assuming the 1 -MW routine beam operation, but we tried further beam loss mitigation to keep the machine activation as low as possible. etp=150 p mm mrad Present : etp=100 p mm mrad ü The foil scattering beam loss can be reduced by larger transverse painting, especially on the horizontal plane. ü But such a large transverse painting had not been realized until recently due to beta function beating caused by the edge focus of the injection bump magnets. Jun 8 th 2015 Average number of foil-hits/particle: ~42 x 0. 6 ~27 17/23 74
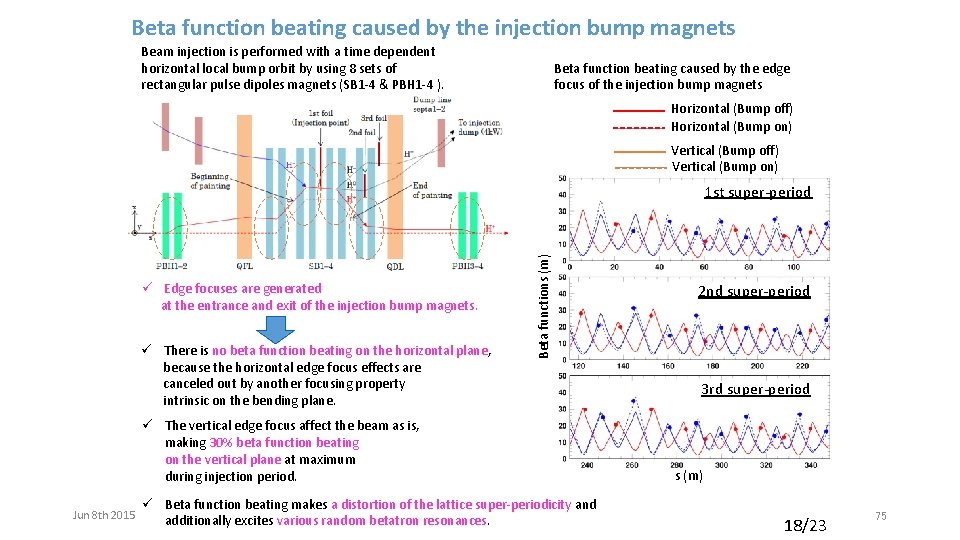
Beta function beating caused by the injection bump magnets Beam injection is performed with a time dependent horizontal local bump orbit by using 8 sets of rectangular pulse dipoles magnets (SB 1 -4 & PBH 1 -4 ). Beta function beating caused by the edge focus of the injection bump magnets Horizontal (Bump off) Horizontal (Bump on) Vertical (Bump off) Vertical (Bump on) ü Edge focuses are generated at the entrance and exit of the injection bump magnets. ü There is no beta function beating on the horizontal plane, because the horizontal edge focus effects are canceled out by another focusing property intrinsic on the bending plane. Beta functions (m) 1 st super-period ü The vertical edge focus affect the beam as is, making 30% beta function beating on the vertical plane at maximum during injection period. Jun 8 th 2015 ü Beta function beating makes a distortion of the lattice super-periodicity and additionally excites various random betatron resonances. 2 nd super-period 3 rd super-period s (m) 18/23 75
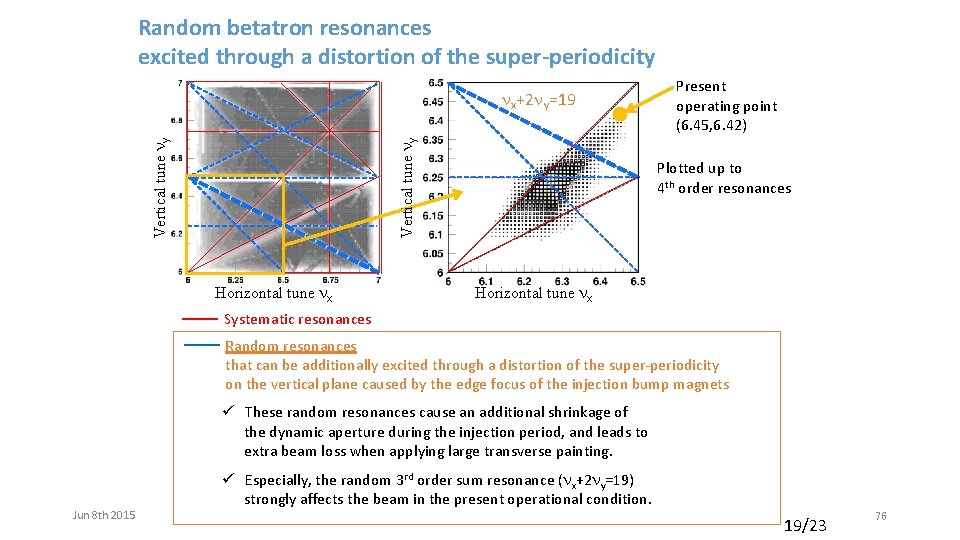
Random betatron resonances excited through a distortion of the super-periodicity Vertical tune ny nx+2 ny=19 Horizontal tune nx Present operating point (6. 45, 6. 42) Plotted up to 4 th order resonances Horizontal tune nx Systematic resonances Random resonances that can be additionally excited through a distortion of the super-periodicity on the vertical plane caused by the edge focus of the injection bump magnets ü These random resonances cause an additional shrinkage of the dynamic aperture during the injection period, and leads to extra beam loss when applying large transverse painting. Jun 8 th 2015 ü Especially, the random 3 rd order sum resonance (nx+2 ny=19) strongly affects the beam in the present operational condition. 19/23 76
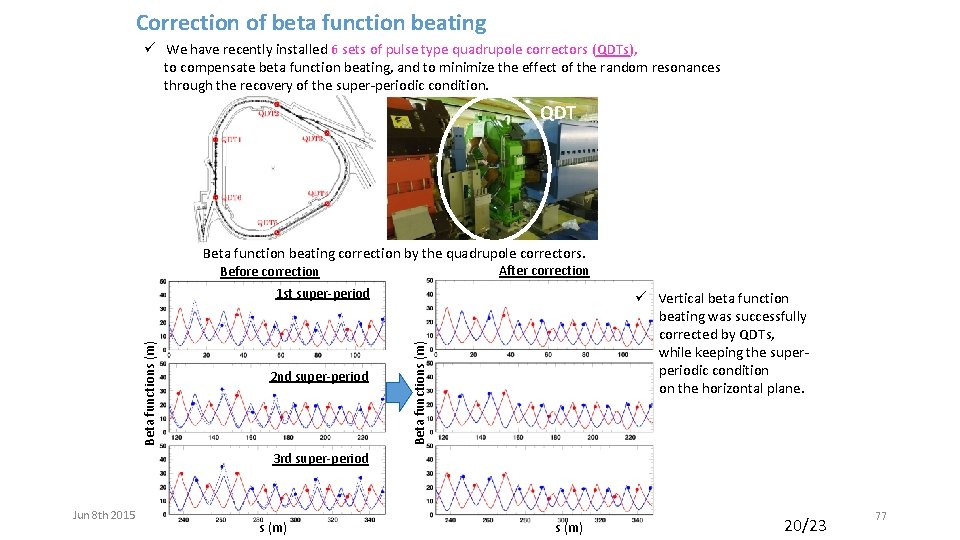
Correction of beta function beating ü We have recently installed 6 sets of pulse type quadrupole correctors (QDTs), to compensate beta function beating, and to minimize the effect of the random resonances through the recovery of the super-periodic condition. QDT Beta function beating correction by the quadrupole correctors. After correction Before correction 2 nd super-period ü Vertical beta function beating was successfully corrected by QDTs, while keeping the superperiodic condition on the horizontal plane. Beta functions (m) 1 st super-period 3 rd super-period Jun 8 th 2015 s (m) 20/23 77
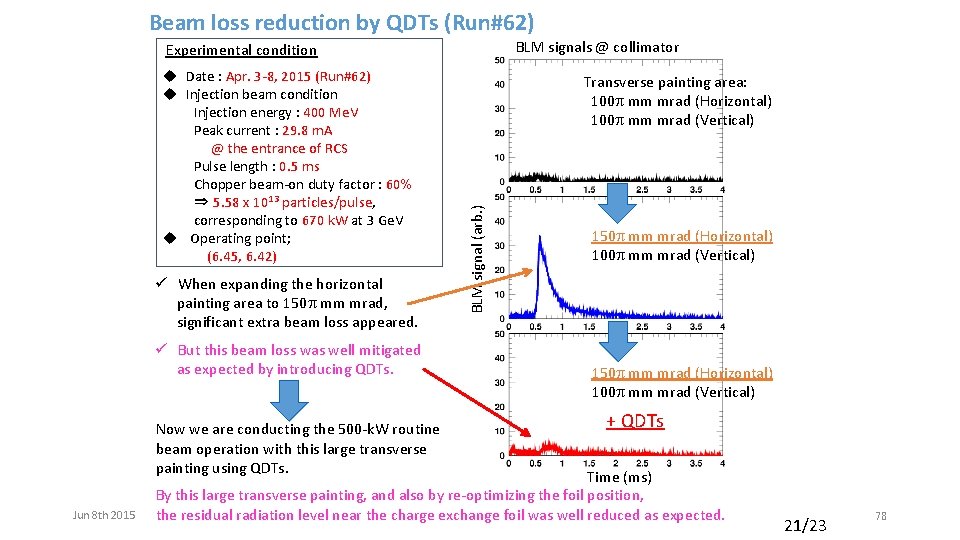
Beam loss reduction by QDTs (Run#62) BLM signals @ collimator Experimental condition ü When expanding the horizontal painting area to 150 p mm mrad, significant extra beam loss appeared. ü But this beam loss was well mitigated as expected by introducing QDTs. Now we are conducting the 500 -k. W routine beam operation with this large transverse painting using QDTs. Jun 8 th 2015 Transverse painting area: 100 p mm mrad (Horizontal) 100 p mm mrad (Vertical) BLM signal (arb. ) u Date : Apr. 3 -8, 2015 (Run#62) u Injection beam condition Injection energy : 400 Me. V Peak current : 29. 8 m. A @ the entrance of RCS Pulse length : 0. 5 ms Chopper beam-on duty factor : 60% ⇒ 5. 58 x 1013 particles/pulse, corresponding to 670 k. W at 3 Ge. V u Operating point; (6. 45, 6. 42) 150 p mm mrad (Horizontal) 100 p mm mrad (Vertical) + QDTs Time (ms) By this large transverse painting, and also by re-optimizing the foil position, the residual radiation level near the charge exchange foil was well reduced as expected. 21/23 78
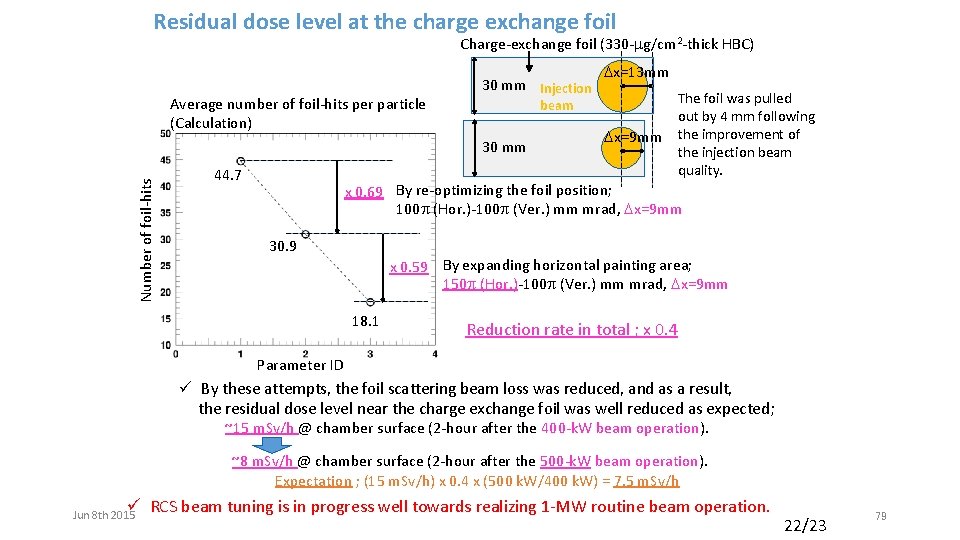
Residual dose level at the charge exchange foil Charge-exchange foil (330 -mg/cm 2 -thick HBC) Average number of foil-hits per particle (Calculation) 30 mm Injection beam Number of foil-hits 30 mm 44. 7 Dx=13 mm Dx=9 mm The foil was pulled out by 4 mm following the improvement of the injection beam quality. x 0. 69 By re-optimizing the foil position; 100 p (Hor. )-100 p (Ver. ) mm mrad, Dx=9 mm 30. 9 x 0. 59 By expanding horizontal painting area; 150 p (Hor. )-100 p (Ver. ) mm mrad, Dx=9 mm 18. 1 Reduction rate in total ; x 0. 4 Parameter ID ü By these attempts, the foil scattering beam loss was reduced, and as a result, the residual dose level near the charge exchange foil was well reduced as expected; ~15 m. Sv/h @ chamber surface (2 -hour after the 400 -k. W beam operation). ~8 m. Sv/h @ chamber surface (2 -hour after the 500 -k. W beam operation). Expectation ; (15 m. Sv/h) x 0. 4 x (500 k. W/400 k. W) = 7. 5 m. Sv/h ü RCS beam tuning is in progress well towards realizing 1 -MW routine beam operation. Jun 8 th 2015 22/23 79
Eduardo harada
Takahiro harada
Harada mori
Urgent care rcs
Inspire rcs
Rcs demo
Rcs electronic control schema elettrico
Rcs+
Rcs japan
Ipod repair rensselaer
Rcs remote control systems
Imprint definition psychology
Early experience vs. later experience
Direct experience and indirect experience
Power system commissioning
Ecs commissioning
Commissioning cycle
Integrated commissioning and progress system
Commissioning
C3sc
Nihr ccf
Fortis furnace commissioning sheet
Croydon clinical commissioning group
Aabc commissioning group
Value based commissioning
Aecp requirements
Commissioning
Timeline jwst
Ecs commissioning
Vacuum systems commissioning
Steps
North west london clinical commissioning group
Pod point commissioning
Commissioning management systems
Experience planning
Food service cycle
Patient experience program
Trinity amputation and prosthesis experience scales
Work experience chart
Radical customer experience
I learned a lot from this experience
Living experience meaning
Lcvp portfolio work experience diary sample
Good judgment comes from
Sips education work experience
Relative experience
It is a verbal representation of a sensory experience
Test taker experience
Adaptive bitrate
Physical activity experience
Effectiveness of nurse residency programs
Contrive experience
Experience
Anchoring and adjustment bias
Ux messen
Preceptorship definition
Veryan work experience
Tncompa
Customer experience management conference 2010
The danish experience
What is numinous
They perfect nature and are perfected by experience
I believe experience
I really enjoyed the experience
Direct experience list
Is an unconscious process created from distilled experience
Life role definition
Design right
Shaping psychology
Experience obeissance
Quality & customer experience forum
Return of experience rex template
Buoutlook
World civilizations the global experience ap edition
Chapter 8: race and ethnicity as lived experience
Learning is a relatively change of behaviors resulting from
Artistic experience
Concrete experience
Cone of experience
Seattle frank gehry