Accelerators for Medicine Maurizio Vretenar CERN 1262018 Academic
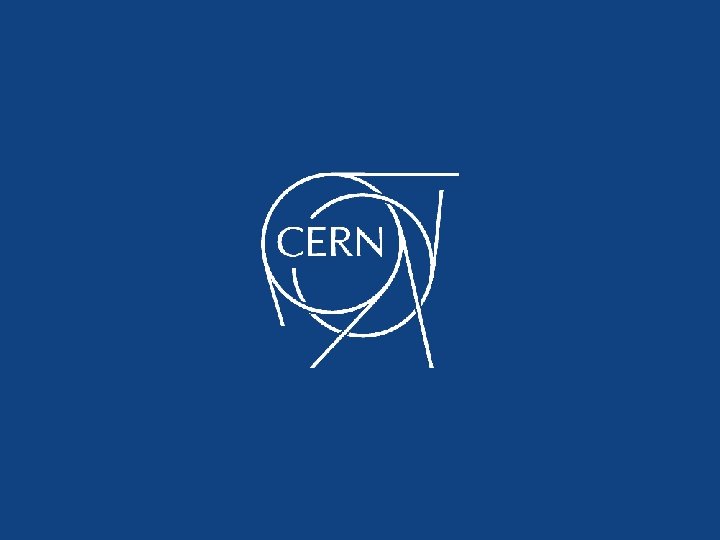
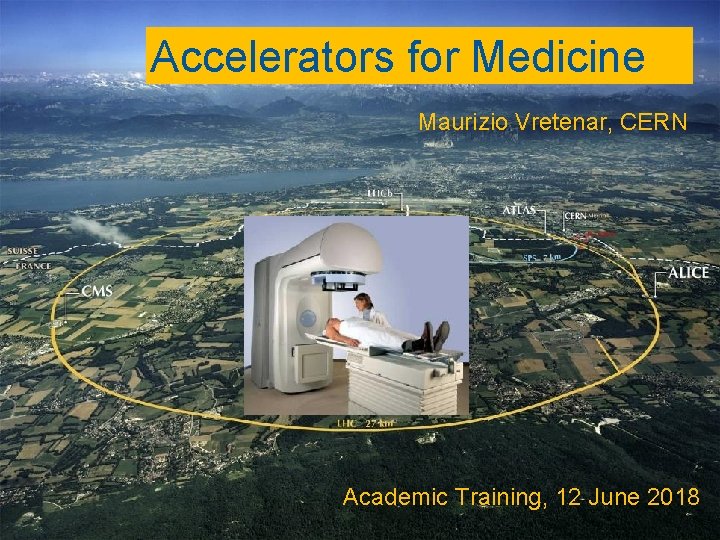
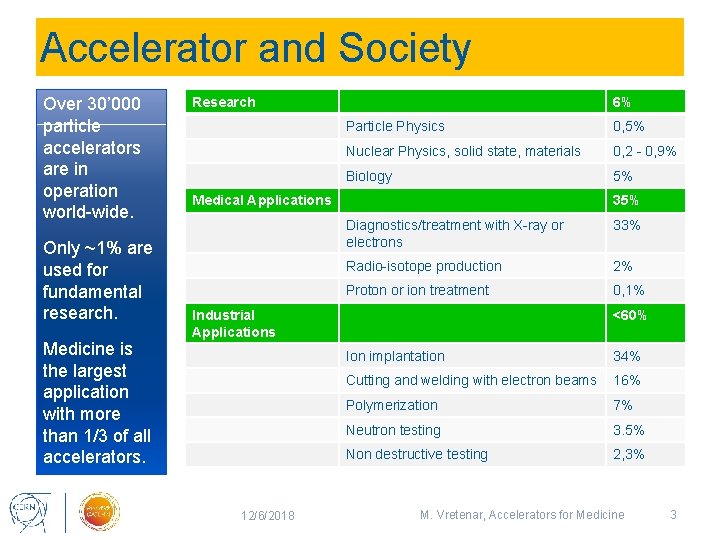
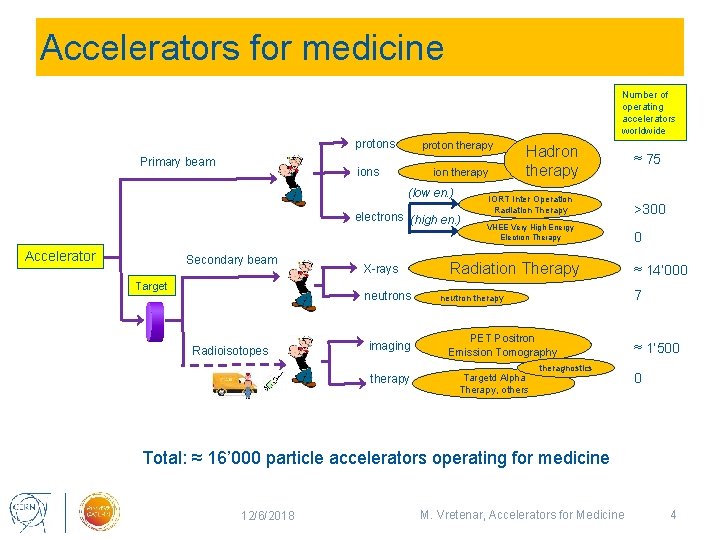
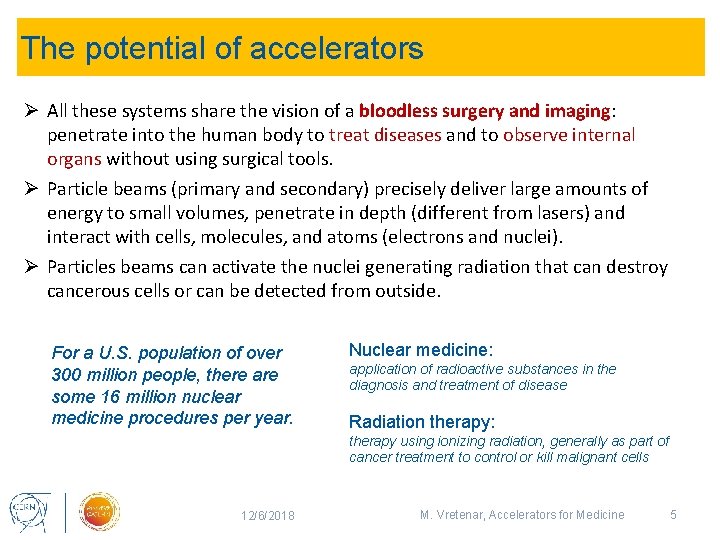
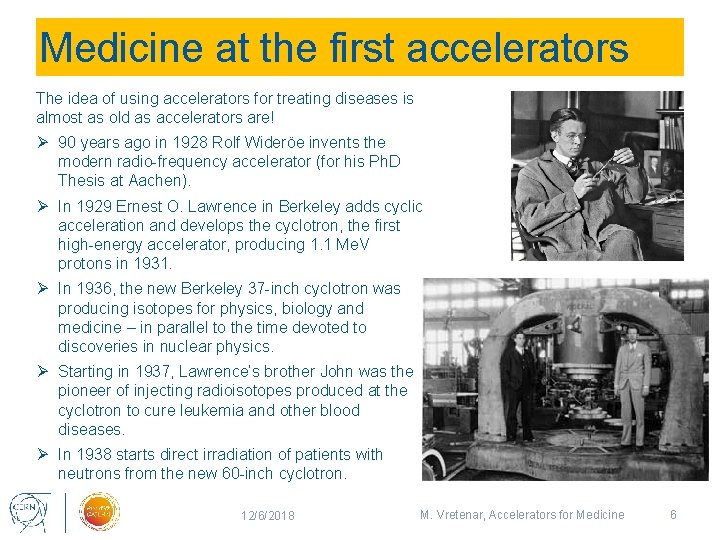
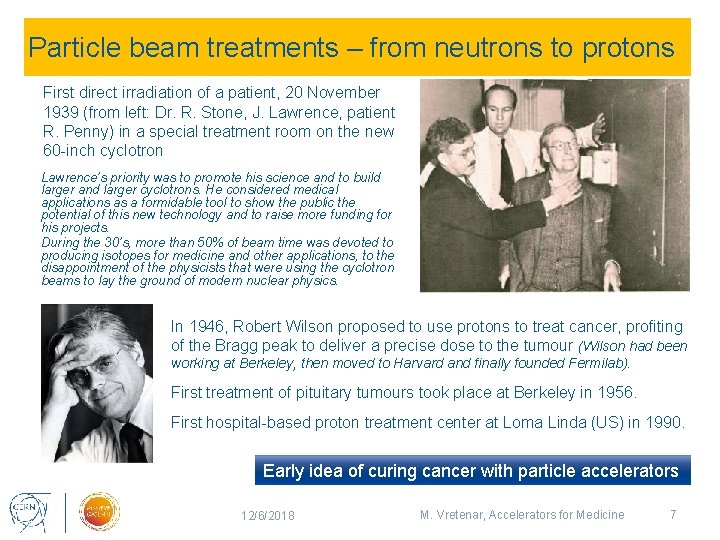
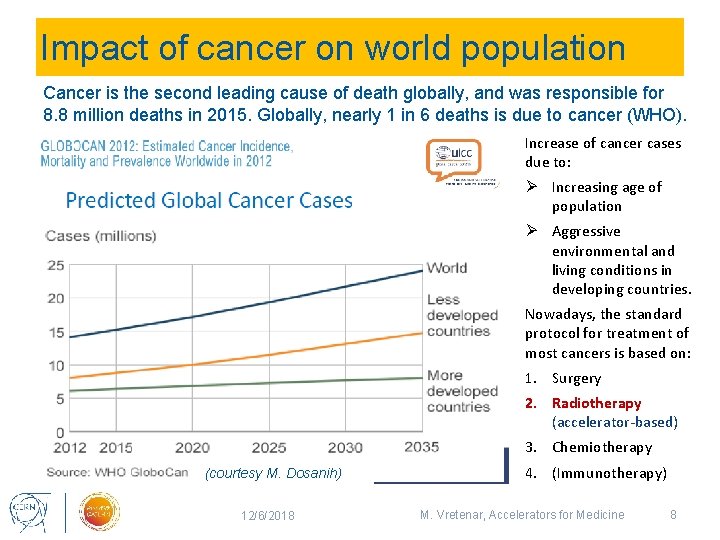
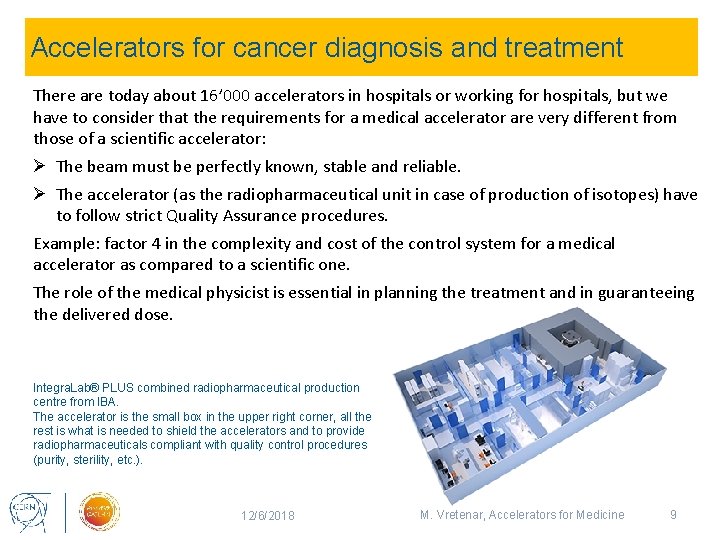
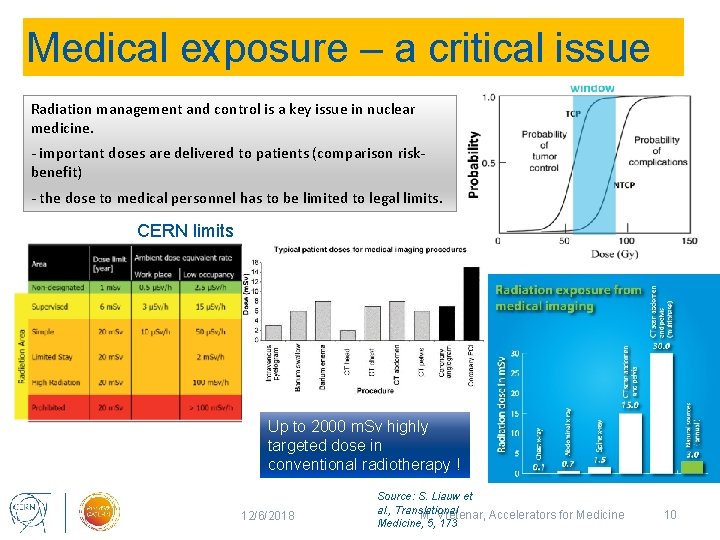
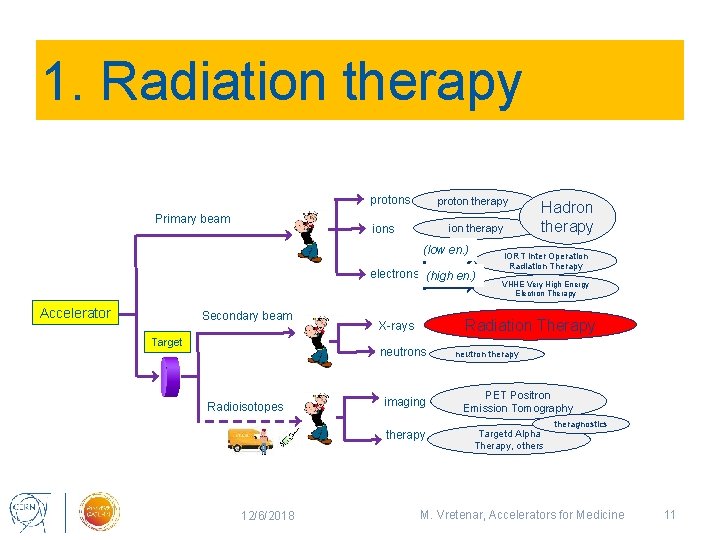
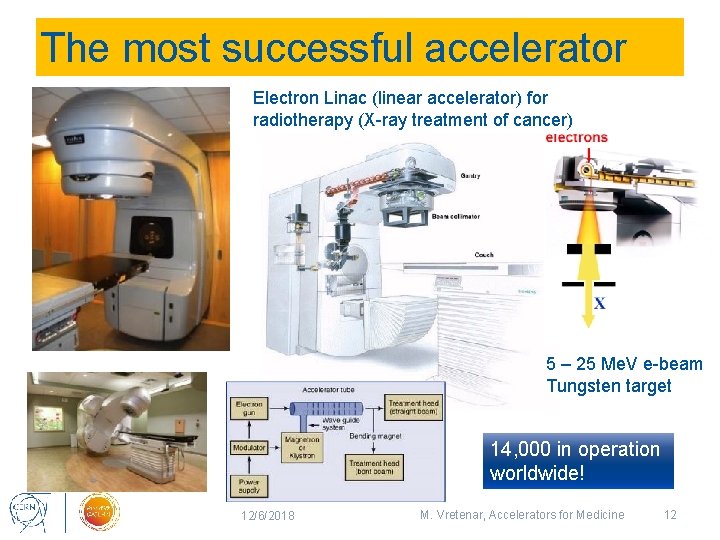
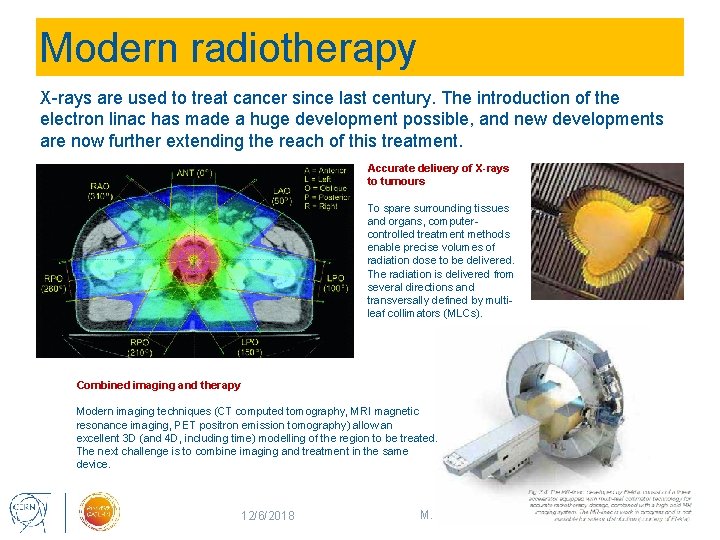
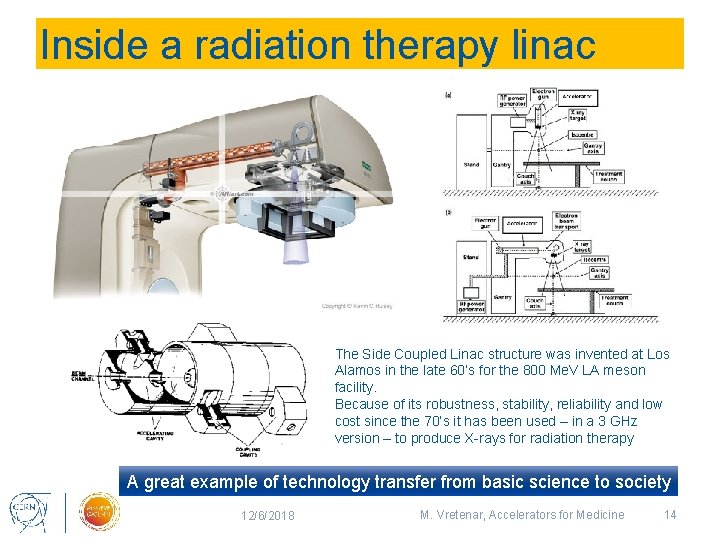
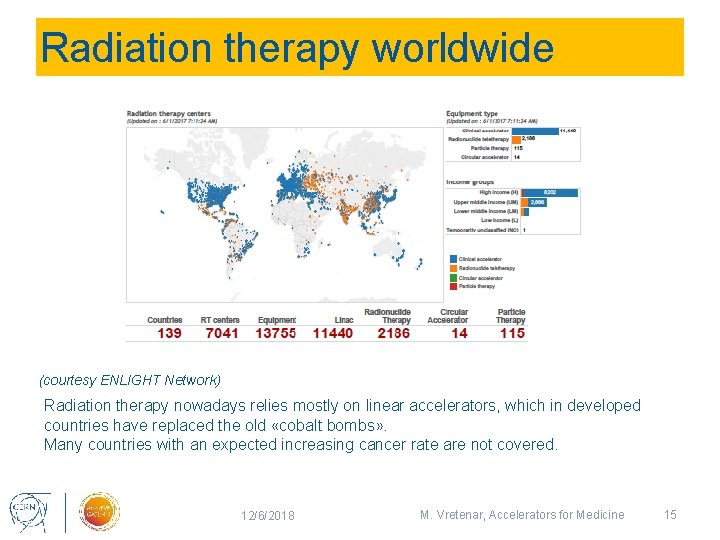
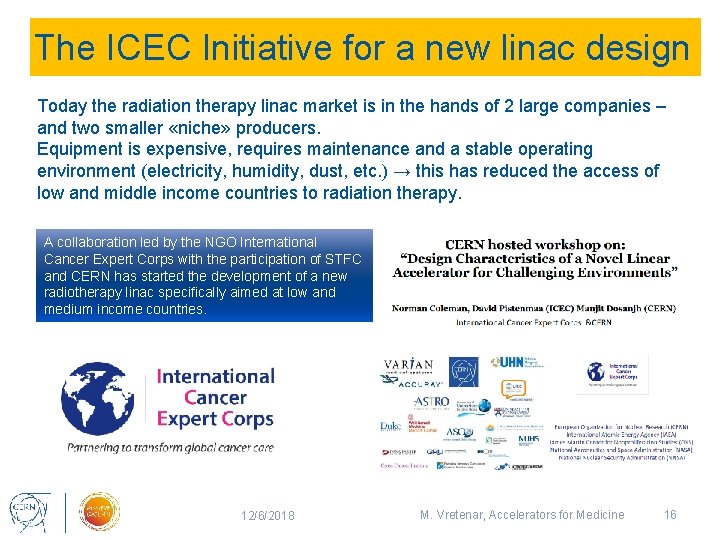
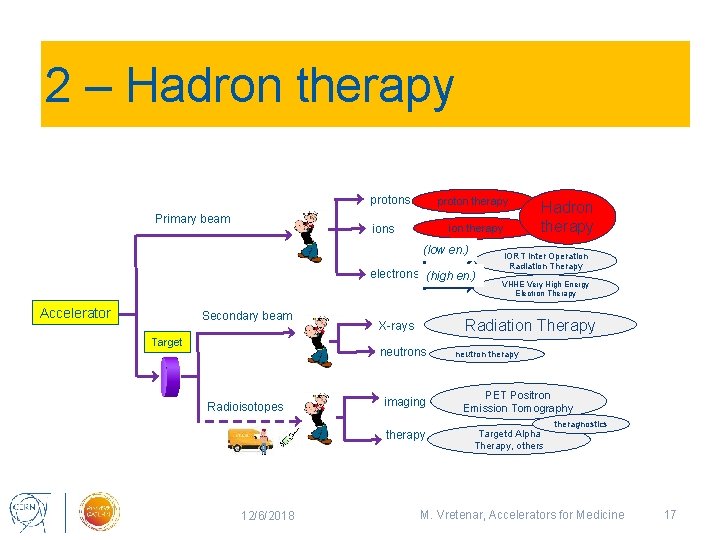
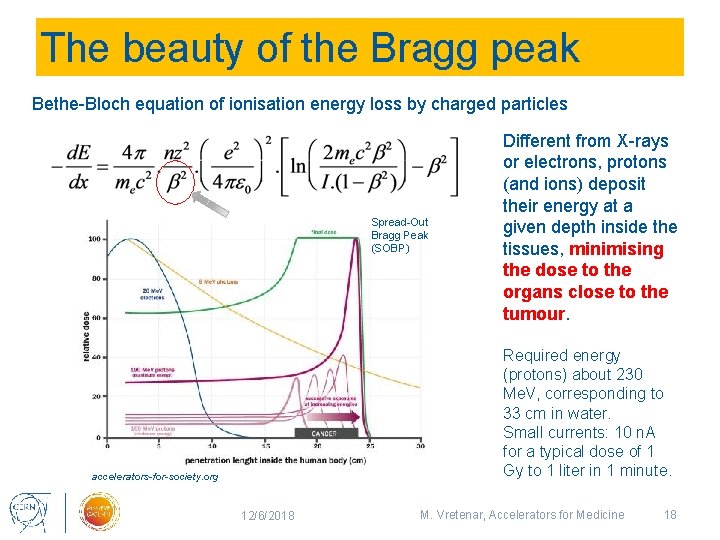
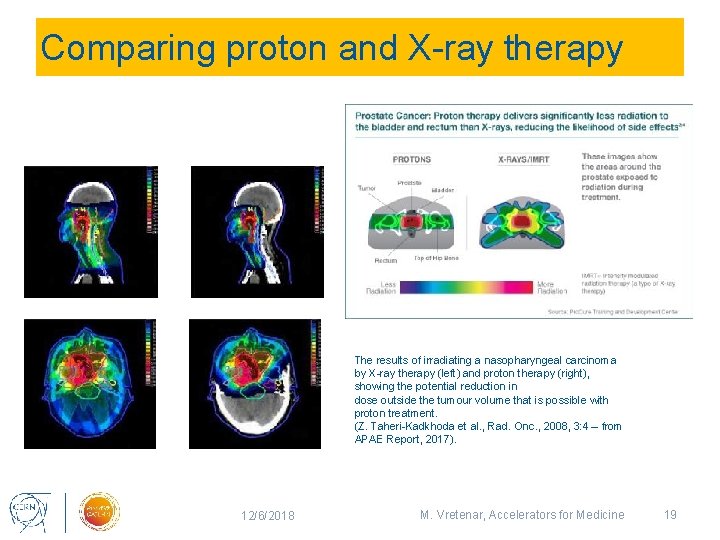
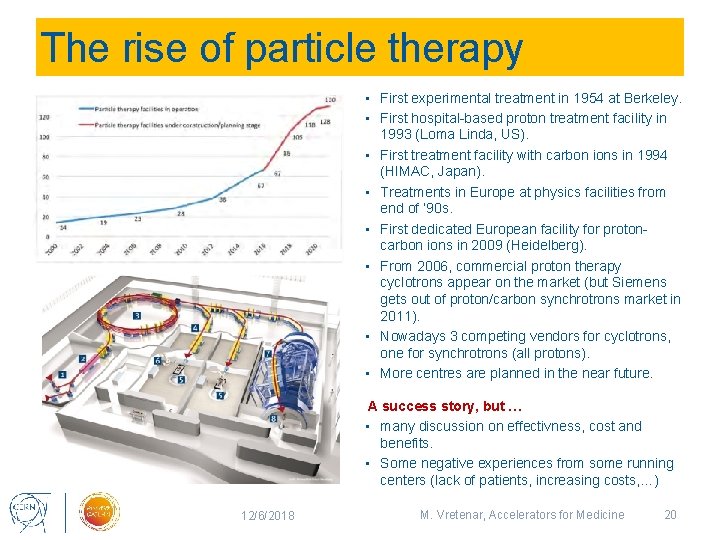
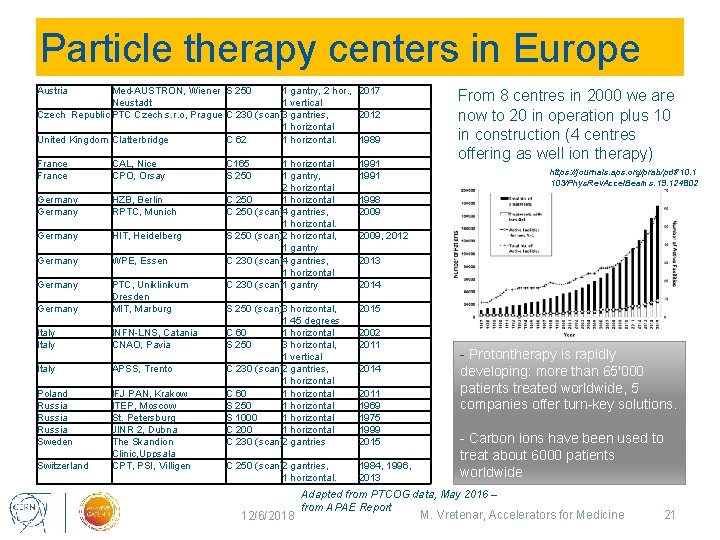
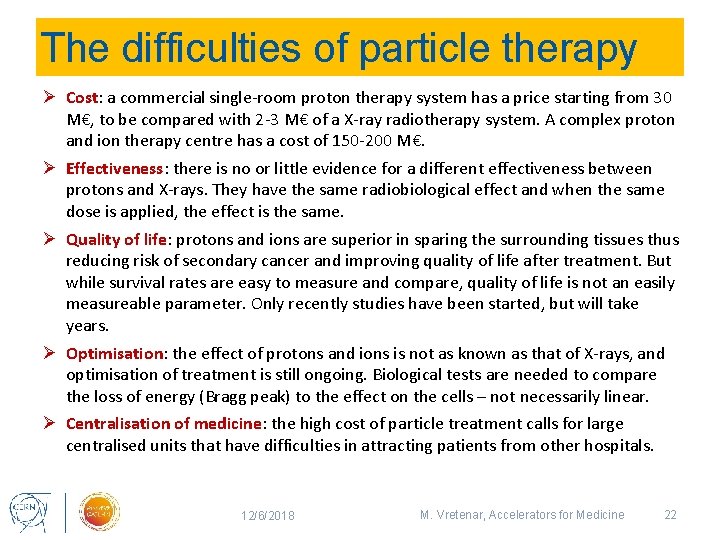
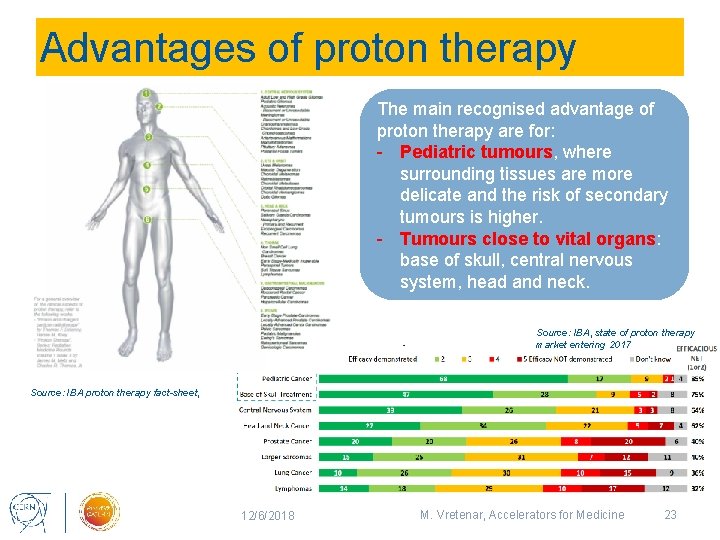
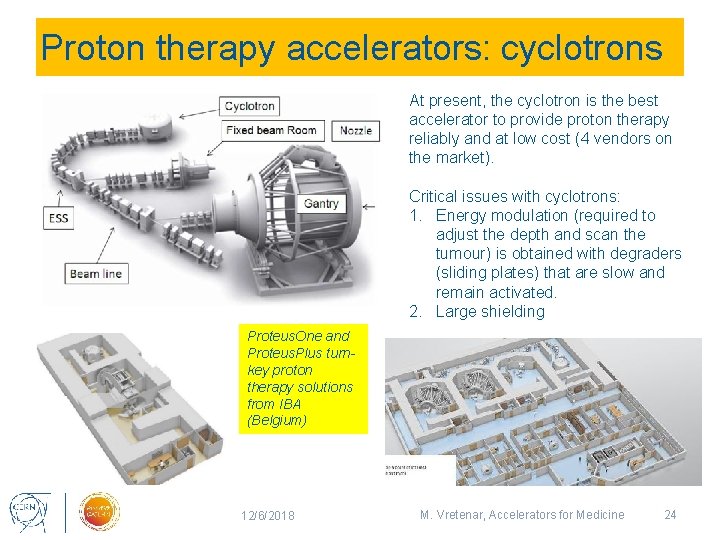
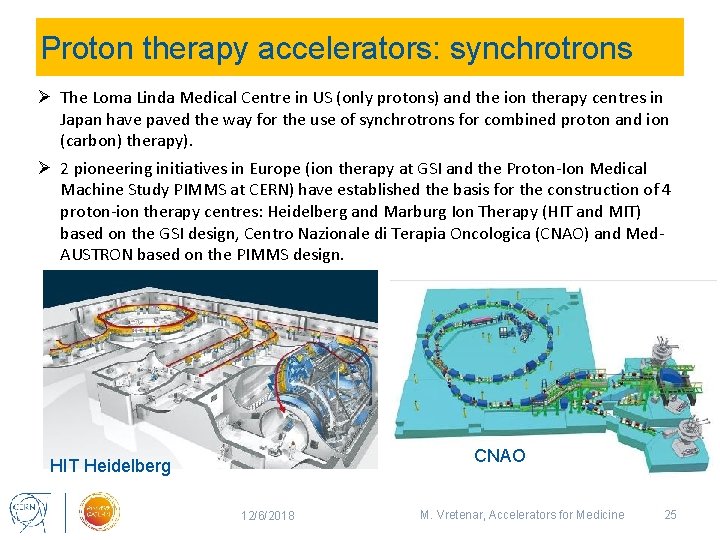
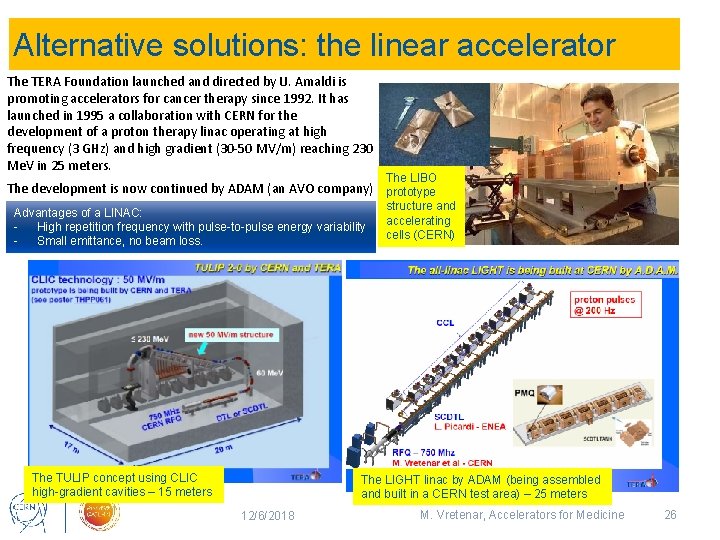
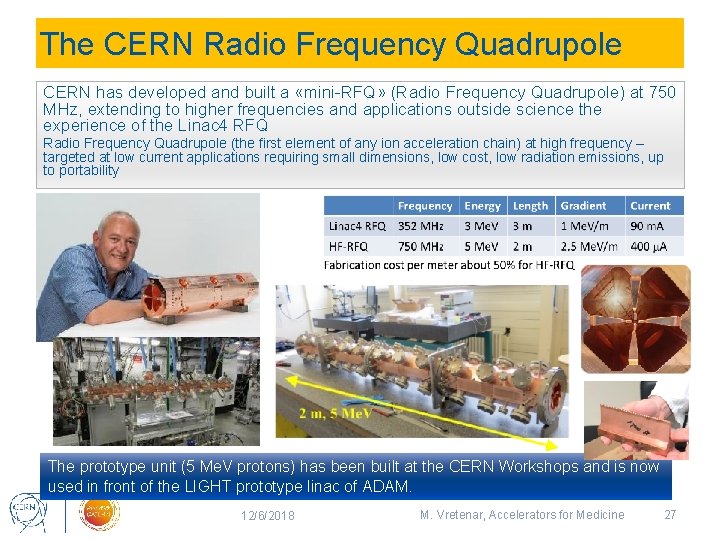
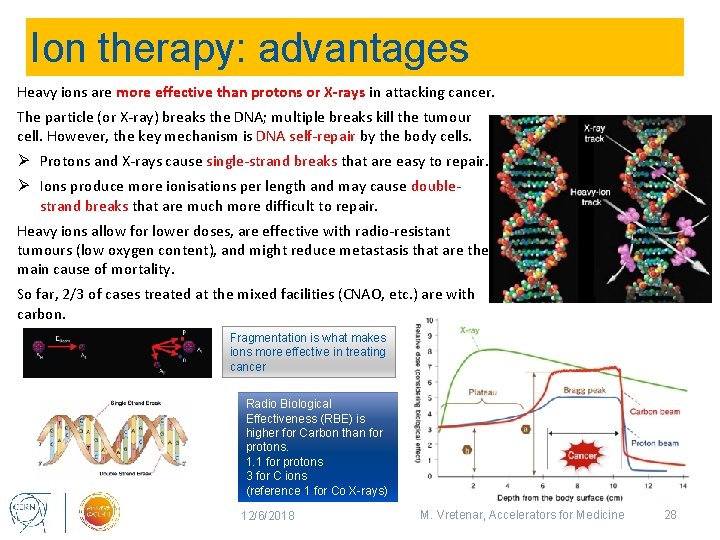
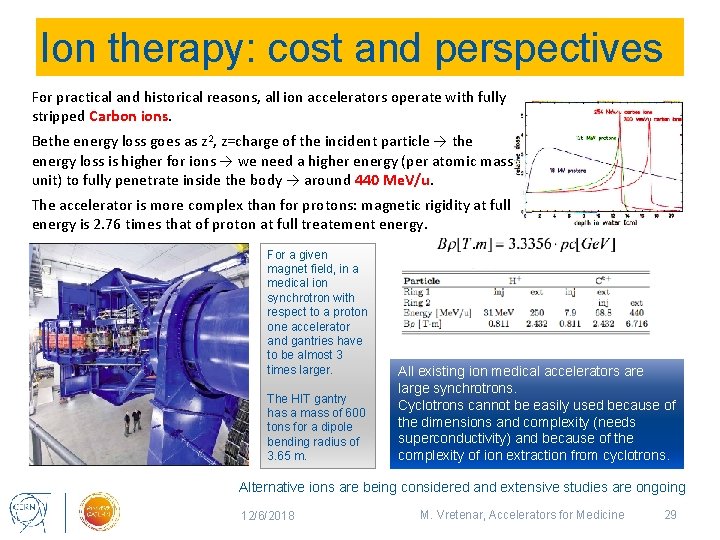
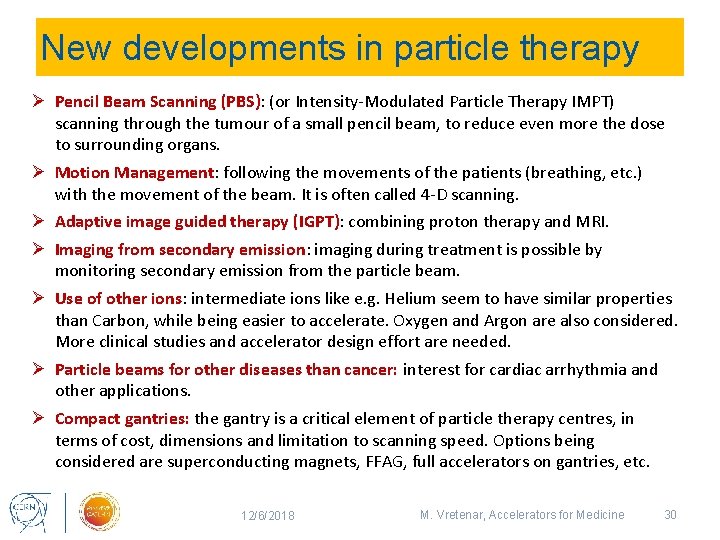
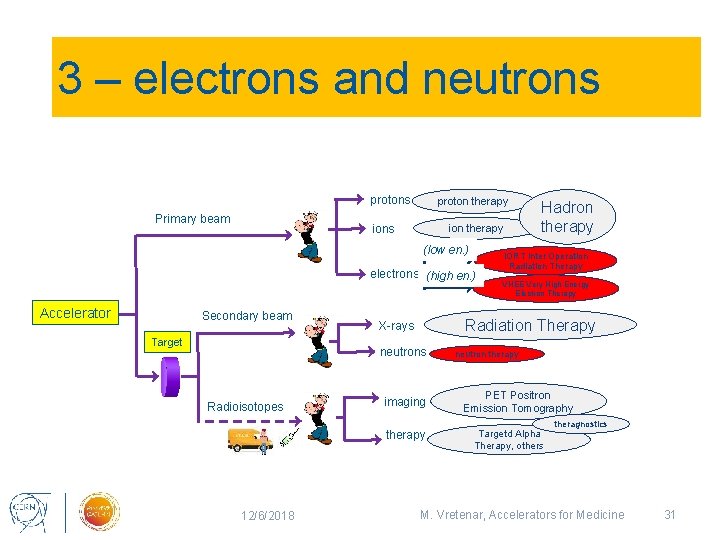
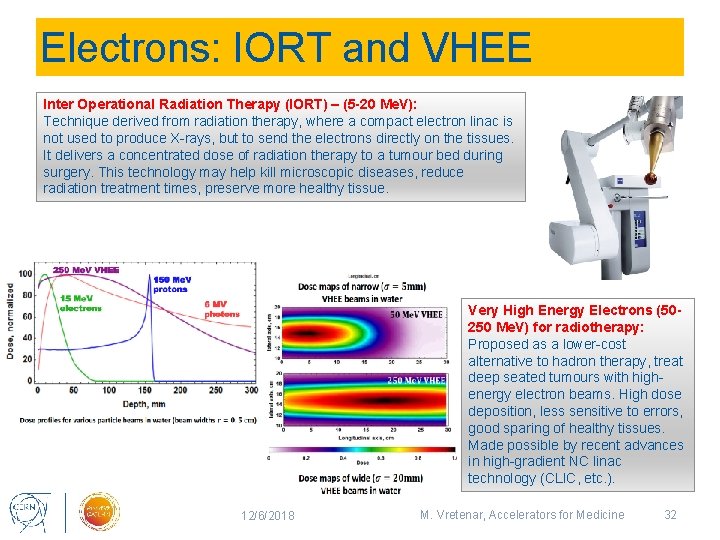
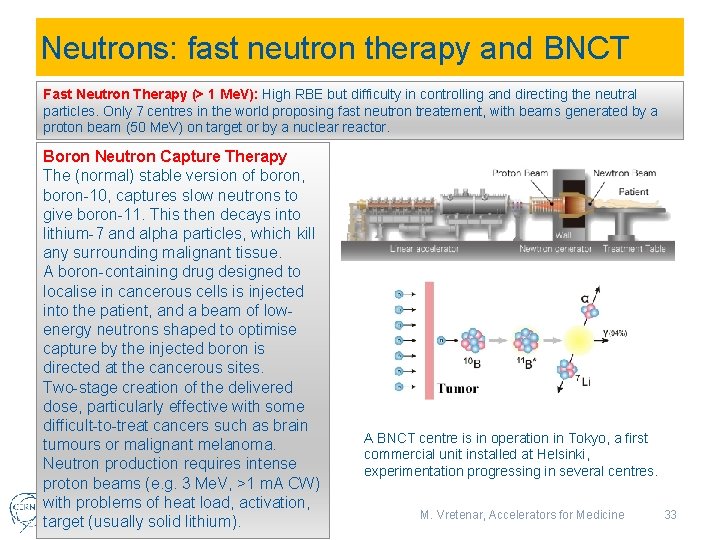
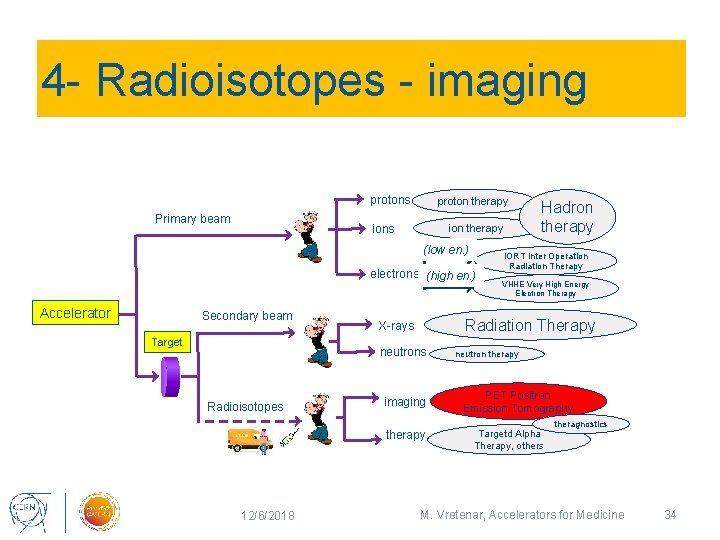
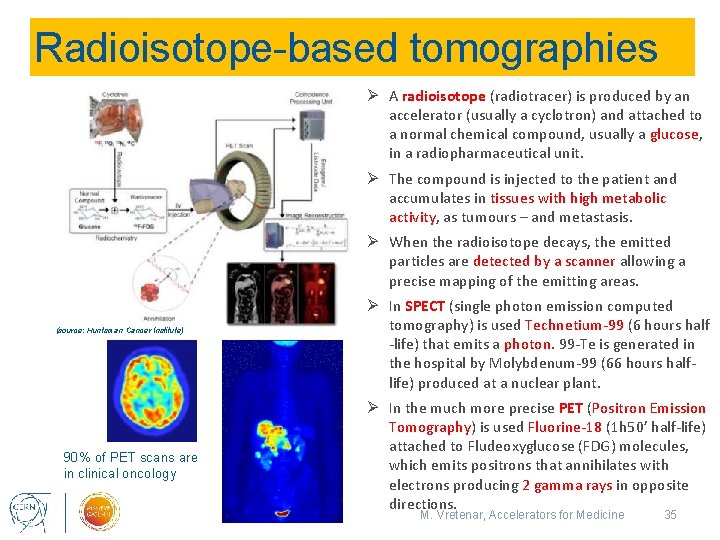
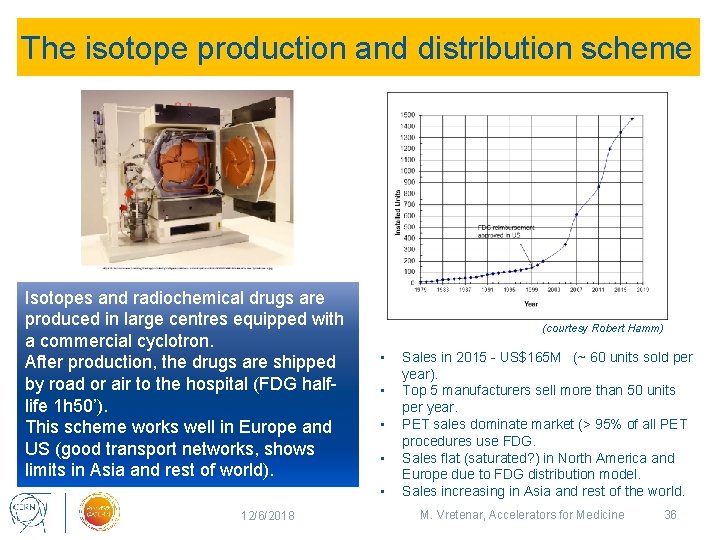
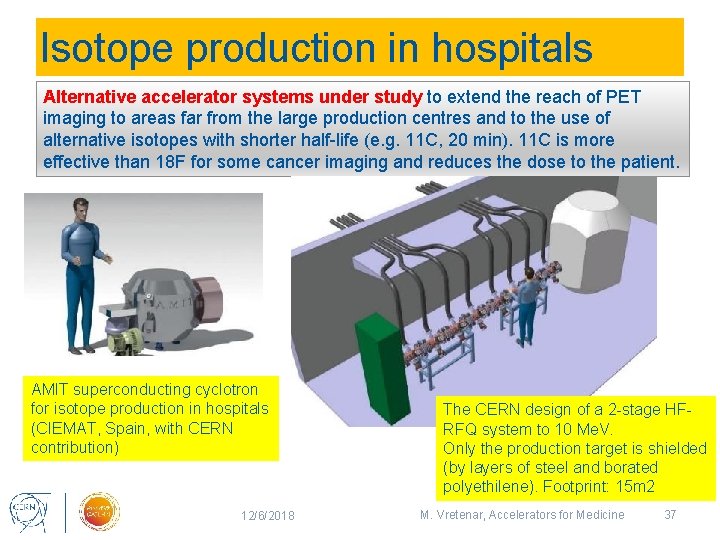
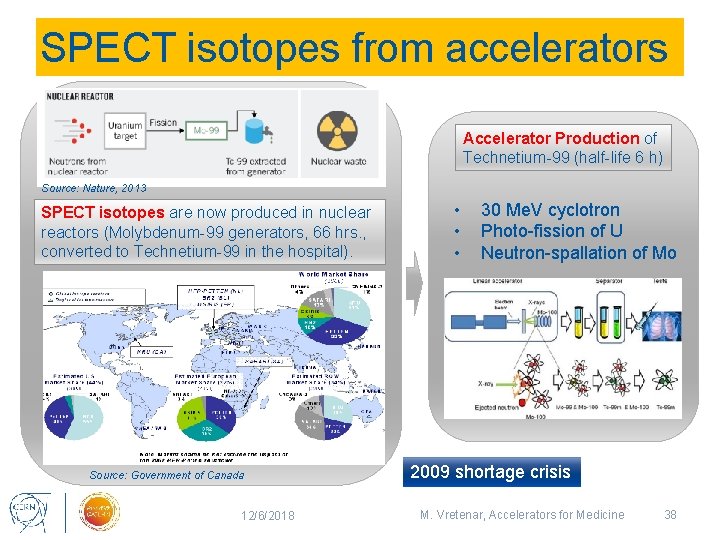
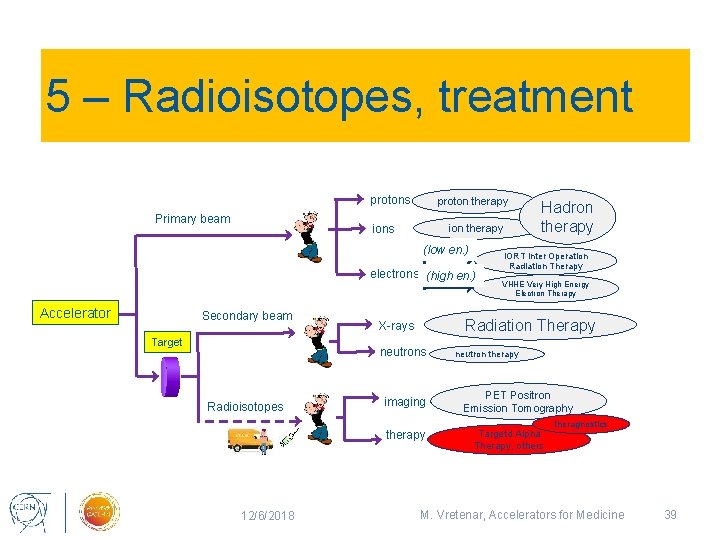
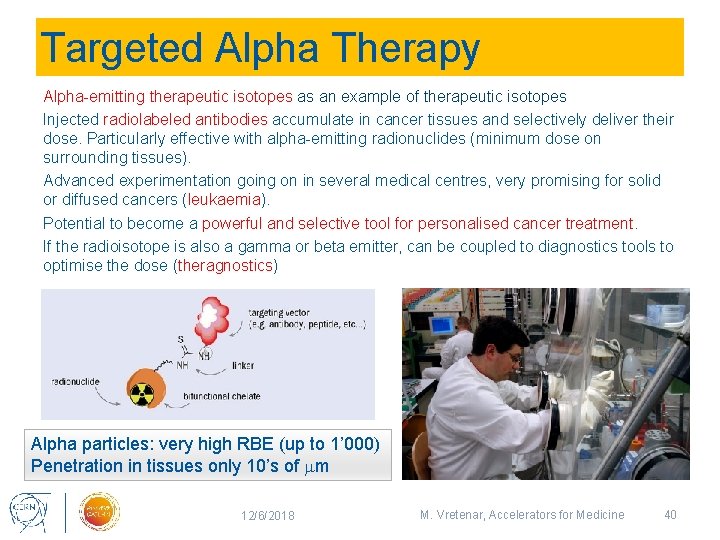
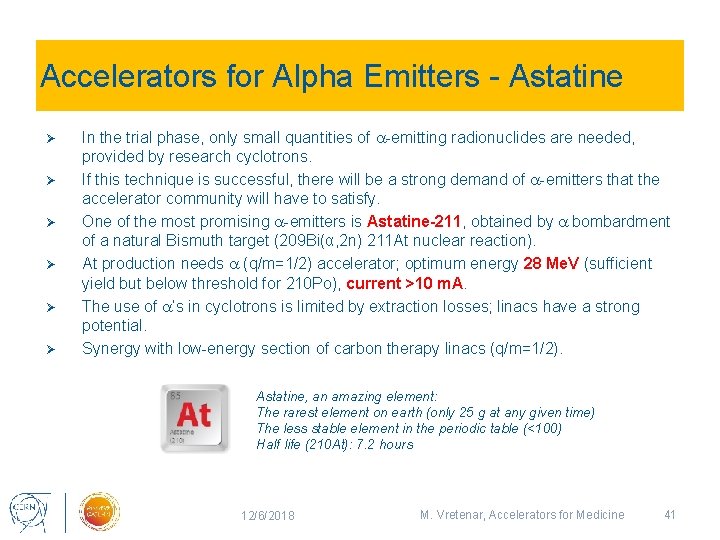
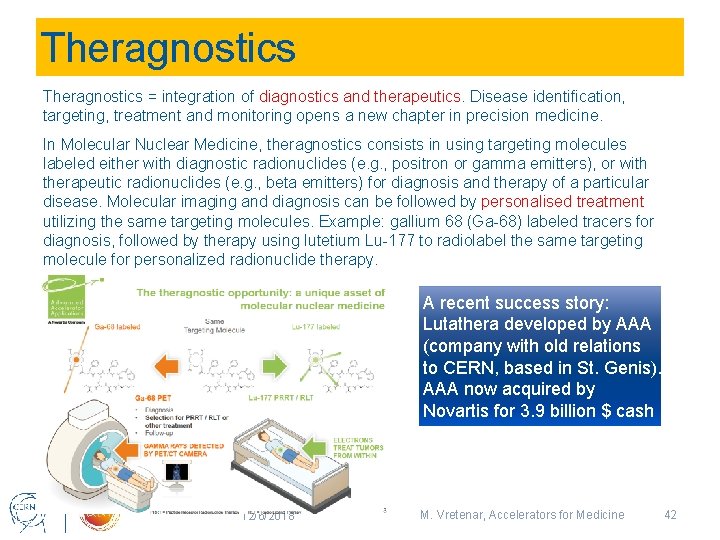
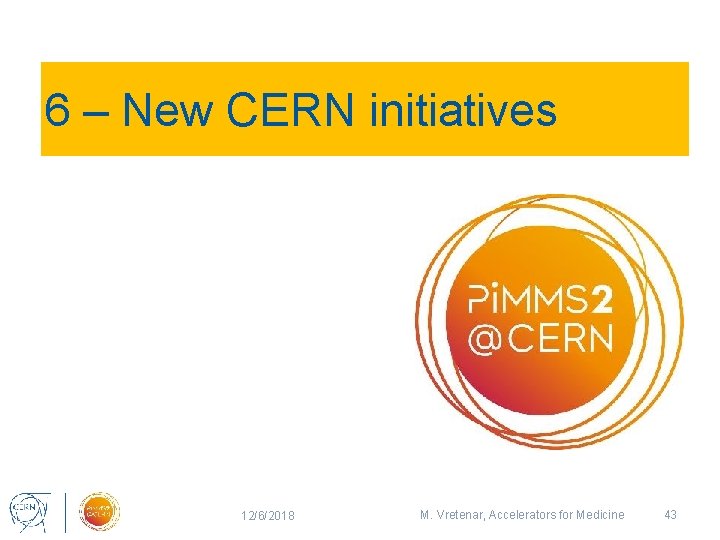
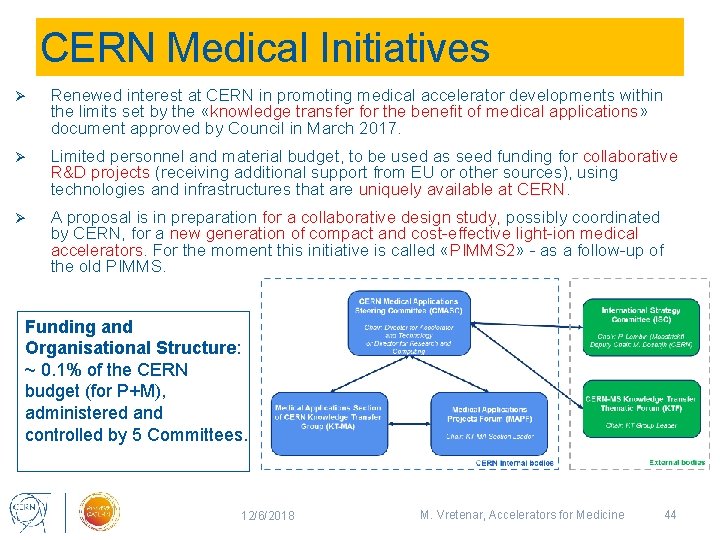
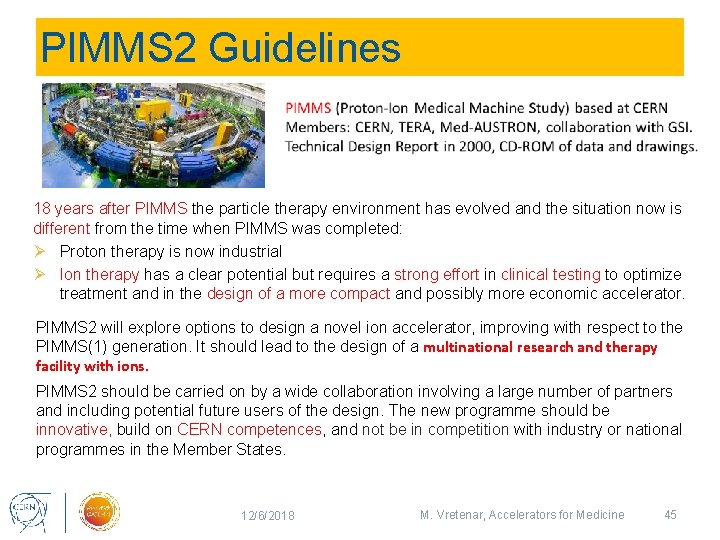
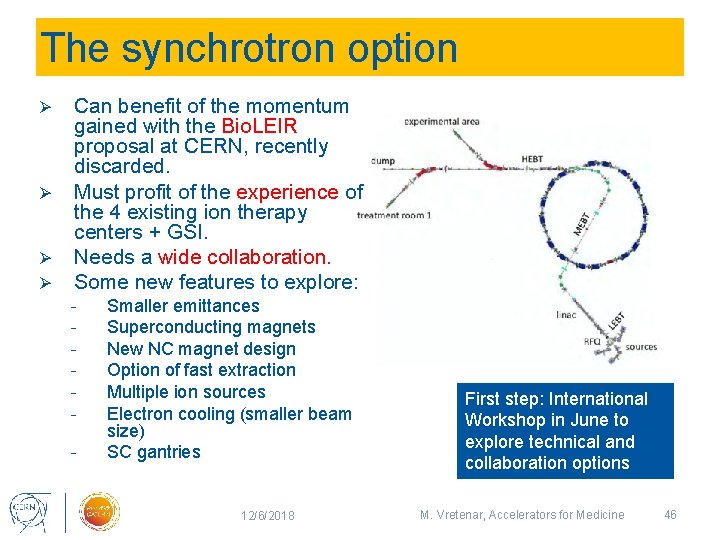
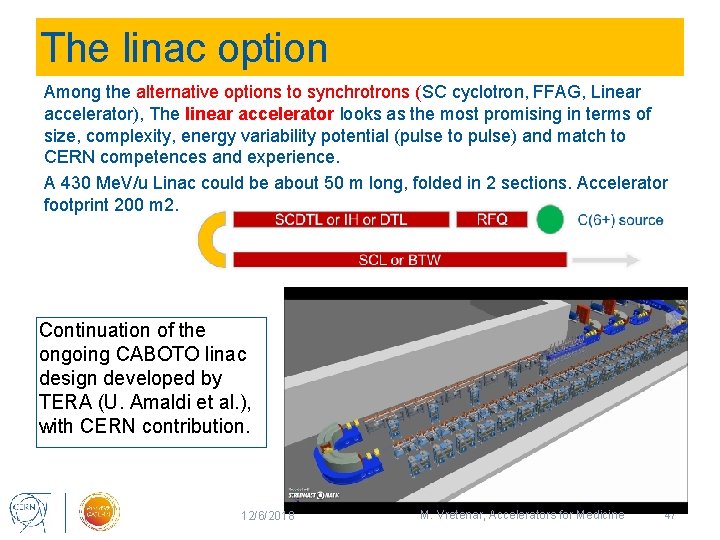
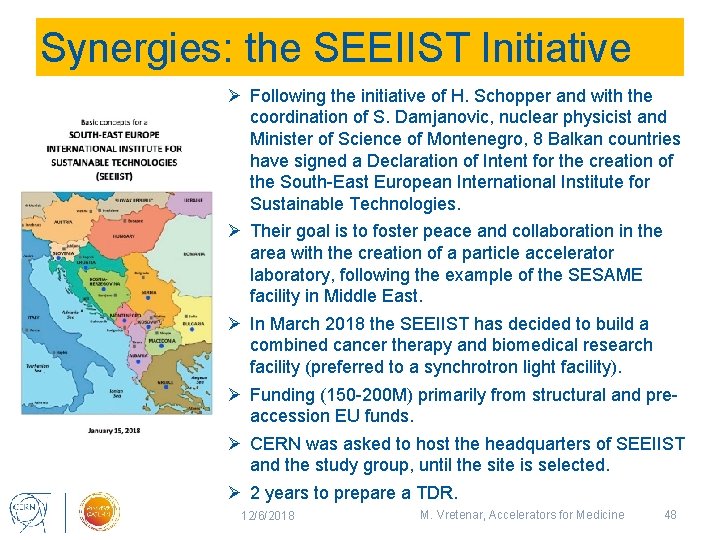
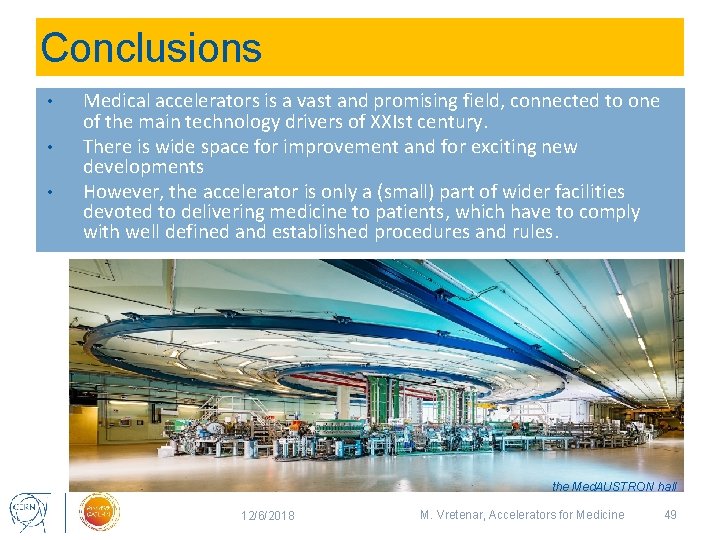
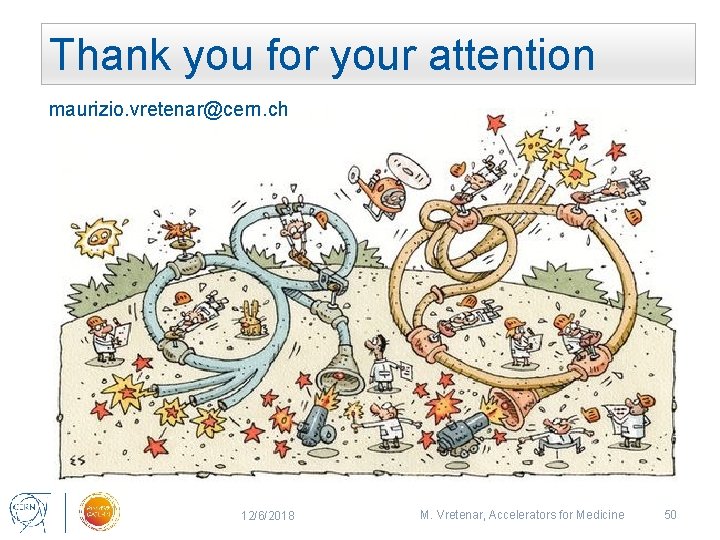
- Slides: 50
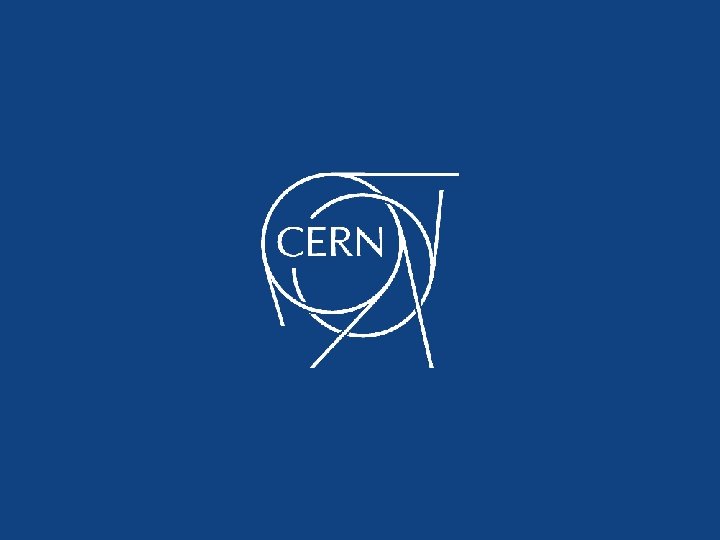
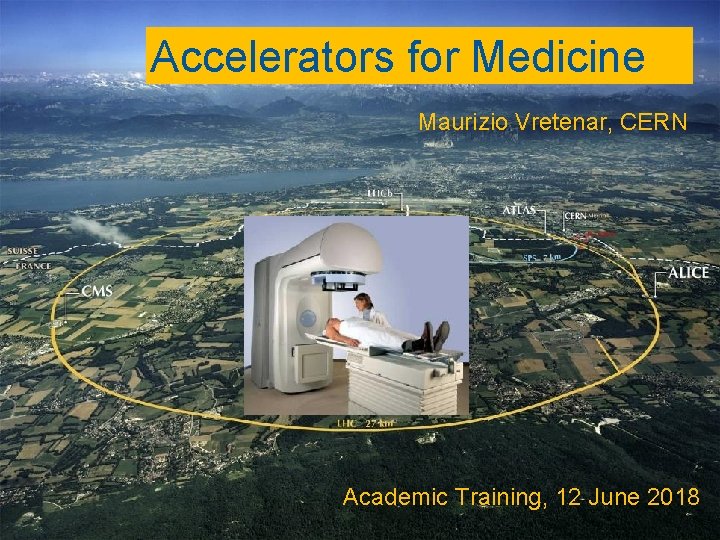
Accelerators for Medicine Maurizio Vretenar, CERN 12/6/2018 Academic Training, 12 June 2018 M. Vretenar, Accelerators for Medicine 2
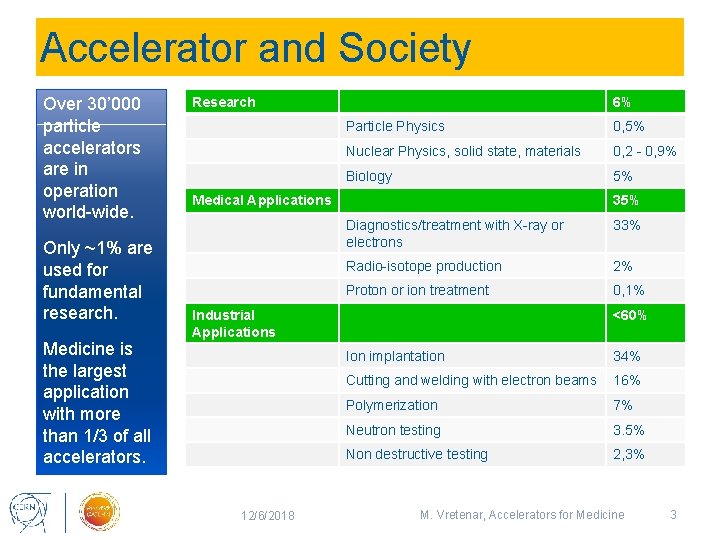
Accelerator and Society Over 30’ 000 particle accelerators are in operation world-wide. Only ~1% are used for fundamental research. Medicine is the largest application with more than 1/3 of all accelerators. Research 6% Particle Physics 0, 5% Nuclear Physics, solid state, materials 0, 2 - 0, 9% Biology 5% Medical Applications 35% Diagnostics/treatment with X-ray or electrons 33% Radio-isotope production 2% Proton or ion treatment 0, 1% Industrial Applications 12/6/2018 <60% Ion implantation 34% Cutting and welding with electron beams 16% Polymerization 7% Neutron testing 3. 5% Non destructive testing 2, 3% M. Vretenar, Accelerators for Medicine 3
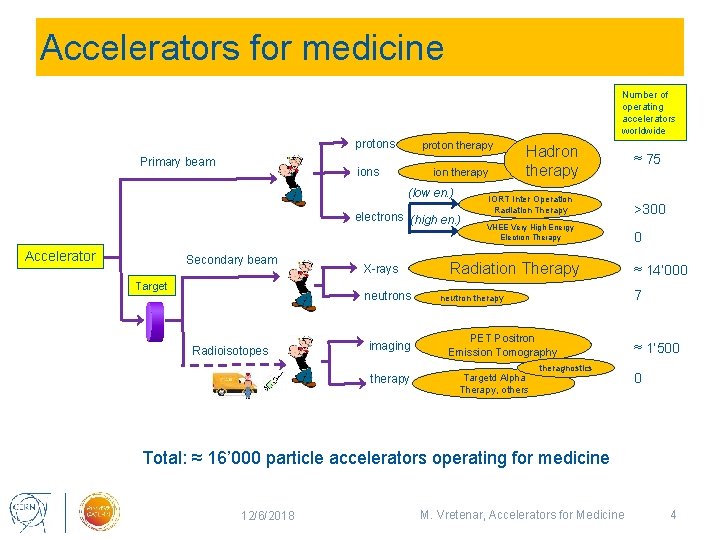
Accelerators for medicine Number of operating accelerators worldwide protons Primary beam proton therapy ions ion therapy (low en. ) electrons (high en. ) Accelerator Secondary beam Target X-rays neutrons Radioisotopes imaging therapy Hadron therapy ≈ 75 IORT Inter Operation Radiation Therapy >300 VHEE Very High Energy Electron Therapy 0 Radiation Therapy 7 neutron therapy PET Positron Emission Tomography Targetd Alpha Therapy, others ≈ 14’ 000 theragnostics ≈ 1’ 500 0 Total: ≈ 16’ 000 particle accelerators operating for medicine 12/6/2018 M. Vretenar, Accelerators for Medicine 4
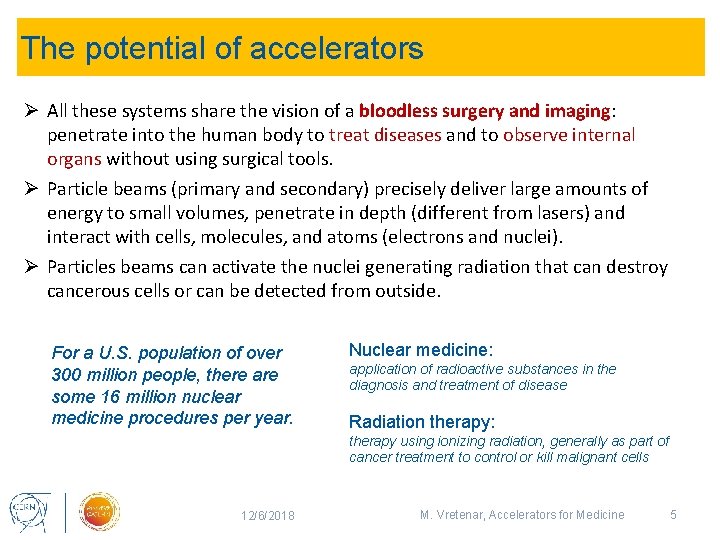
The potential of accelerators Ø All these systems share the vision of a bloodless surgery and imaging: penetrate into the human body to treat diseases and to observe internal organs without using surgical tools. Ø Particle beams (primary and secondary) precisely deliver large amounts of energy to small volumes, penetrate in depth (different from lasers) and interact with cells, molecules, and atoms (electrons and nuclei). Ø Particles beams can activate the nuclei generating radiation that can destroy cancerous cells or can be detected from outside. For a U. S. population of over 300 million people, there are some 16 million nuclear medicine procedures per year. Nuclear medicine: application of radioactive substances in the diagnosis and treatment of disease Radiation therapy: therapy using ionizing radiation, generally as part of cancer treatment to control or kill malignant cells 12/6/2018 M. Vretenar, Accelerators for Medicine 5
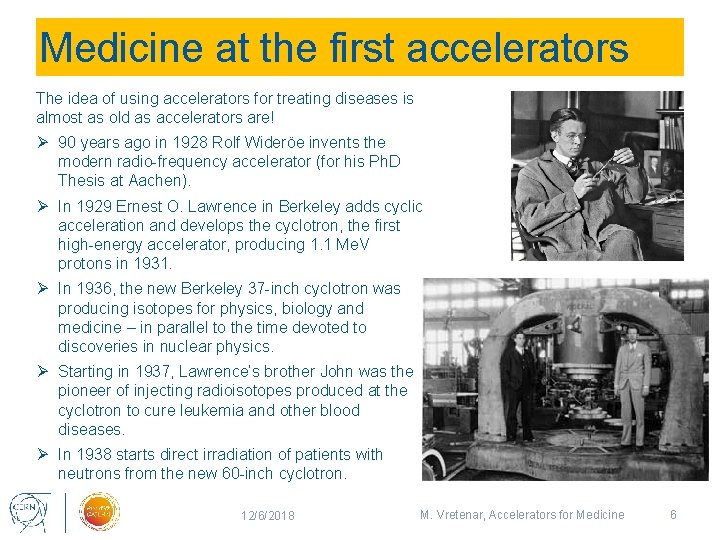
Medicine at the first accelerators The idea of using accelerators for treating diseases is almost as old as accelerators are! Ø 90 years ago in 1928 Rolf Wideröe invents the modern radio-frequency accelerator (for his Ph. D Thesis at Aachen). Ø In 1929 Ernest O. Lawrence in Berkeley adds cyclic acceleration and develops the cyclotron, the first high-energy accelerator, producing 1. 1 Me. V protons in 1931. Ø In 1936, the new Berkeley 37 -inch cyclotron was producing isotopes for physics, biology and medicine – in parallel to the time devoted to discoveries in nuclear physics. Ø Starting in 1937, Lawrence’s brother John was the pioneer of injecting radioisotopes produced at the cyclotron to cure leukemia and other blood diseases. Ø In 1938 starts direct irradiation of patients with neutrons from the new 60 -inch cyclotron. 12/6/2018 M. Vretenar, Accelerators for Medicine 6
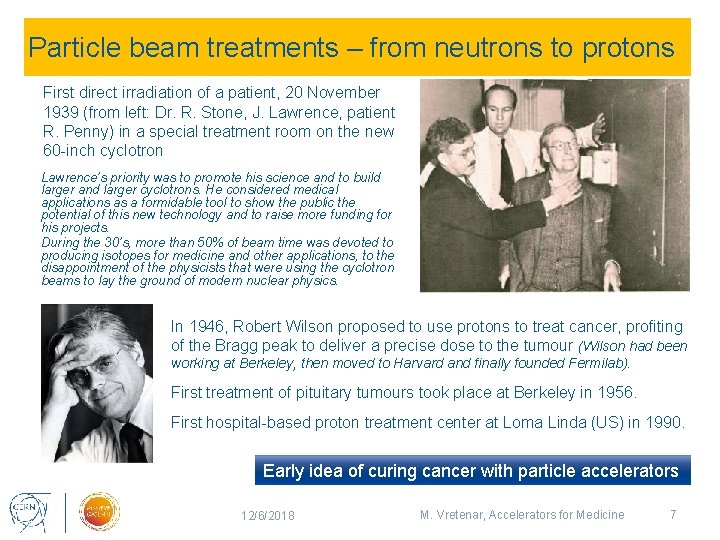
Particle beam treatments – from neutrons to protons First direct irradiation of a patient, 20 November 1939 (from left: Dr. R. Stone, J. Lawrence, patient R. Penny) in a special treatment room on the new 60 -inch cyclotron Lawrence’s priority was to promote his science and to build larger and larger cyclotrons. He considered medical applications as a formidable tool to show the public the potential of this new technology and to raise more funding for his projects. During the 30’s, more than 50% of beam time was devoted to producing isotopes for medicine and other applications, to the disappointment of the physicists that were using the cyclotron beams to lay the ground of modern nuclear physics. In 1946, Robert Wilson proposed to use protons to treat cancer, profiting of the Bragg peak to deliver a precise dose to the tumour (Wilson had been working at Berkeley, then moved to Harvard and finally founded Fermilab). First treatment of pituitary tumours took place at Berkeley in 1956. First hospital-based proton treatment center at Loma Linda (US) in 1990. Early idea of curing cancer with particle accelerators 12/6/2018 M. Vretenar, Accelerators for Medicine 7
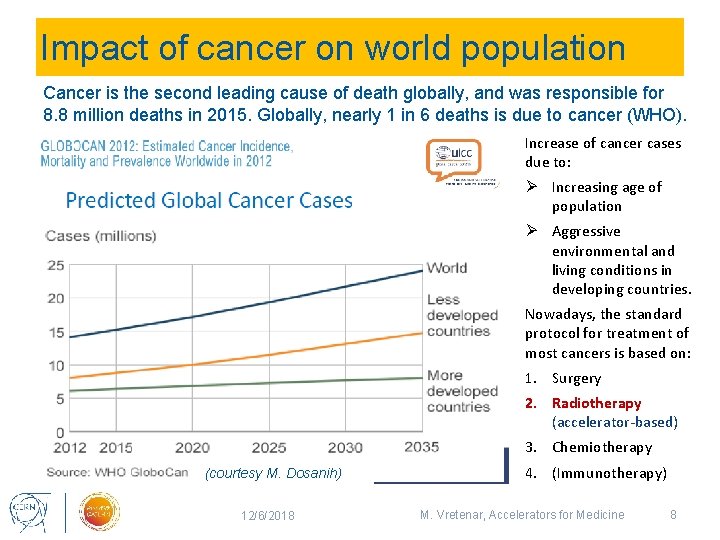
Impact of cancer on world population Cancer is the second leading cause of death globally, and was responsible for 8. 8 million deaths in 2015. Globally, nearly 1 in 6 deaths is due to cancer (WHO). Increase of cancer cases due to: Ø Increasing age of population Ø Aggressive environmental and living conditions in developing countries. Nowadays, the standard protocol for treatment of most cancers is based on: 1. Surgery 2. Radiotherapy (accelerator-based) 3. Chemiotherapy (courtesy M. Dosanih) 12/6/2018 4. (Immunotherapy) M. Vretenar, Accelerators for Medicine 8
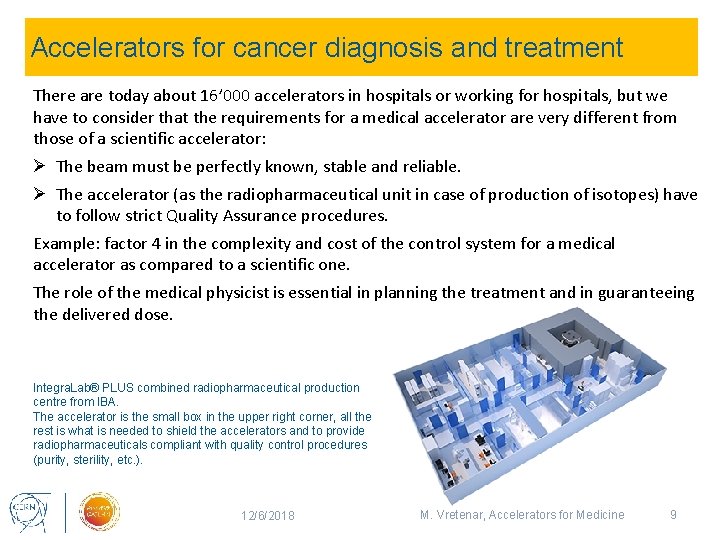
Accelerators for cancer diagnosis and treatment There are today about 16’ 000 accelerators in hospitals or working for hospitals, but we have to consider that the requirements for a medical accelerator are very different from those of a scientific accelerator: Ø The beam must be perfectly known, stable and reliable. Ø The accelerator (as the radiopharmaceutical unit in case of production of isotopes) have to follow strict Quality Assurance procedures. Example: factor 4 in the complexity and cost of the control system for a medical accelerator as compared to a scientific one. The role of the medical physicist is essential in planning the treatment and in guaranteeing the delivered dose. Integra. Lab® PLUS combined radiopharmaceutical production centre from IBA. The accelerator is the small box in the upper right corner, all the rest is what is needed to shield the accelerators and to provide radiopharmaceuticals compliant with quality control procedures (purity, sterility, etc. ). 12/6/2018 M. Vretenar, Accelerators for Medicine 9
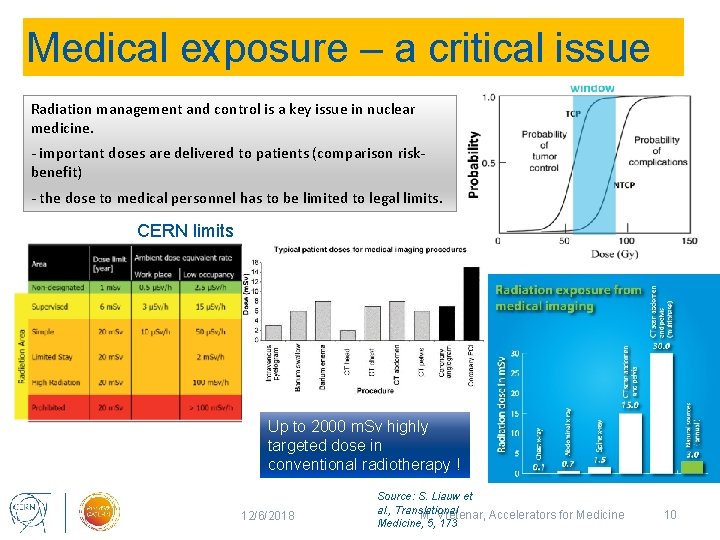
Medical exposure – a critical issue Radiation management and control is a key issue in nuclear medicine. - important doses are delivered to patients (comparison riskbenefit) - the dose to medical personnel has to be limited to legal limits. CERN limits Up to 2000 m. Sv highly targeted dose in conventional radiotherapy ! 12/6/2018 Source: S. Liauw et al. , Translational M. Vretenar, Accelerators for Medicine, 5, 173 10
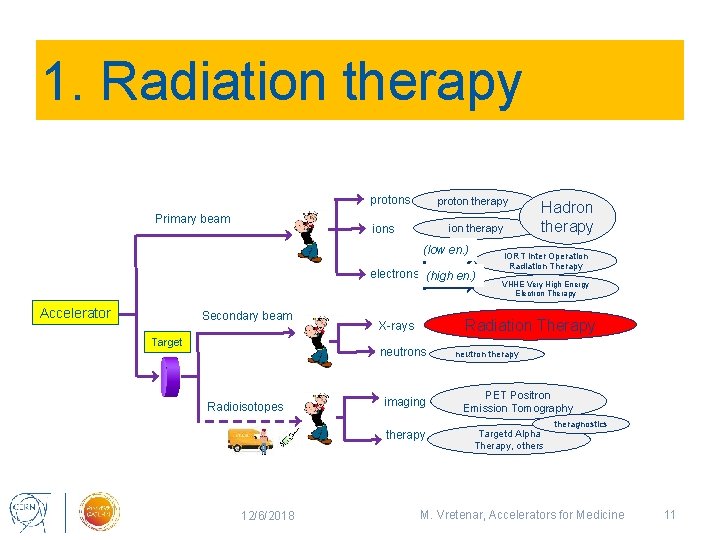
1. Radiation therapy protons Primary beam proton therapy ions ion therapy (low en. ) electrons (high en. ) Accelerator Secondary beam Target imaging therapy 12/6/2018 IORT Inter Operation Radiation Therapy VHHE Very High Energy Electron Therapy Radiation Therapy X-rays neutrons Radioisotopes Hadron therapy neutron therapy PET Positron Emission Tomography Targetd Alpha Therapy, others theragnostics M. Vretenar, Accelerators for Medicine 11
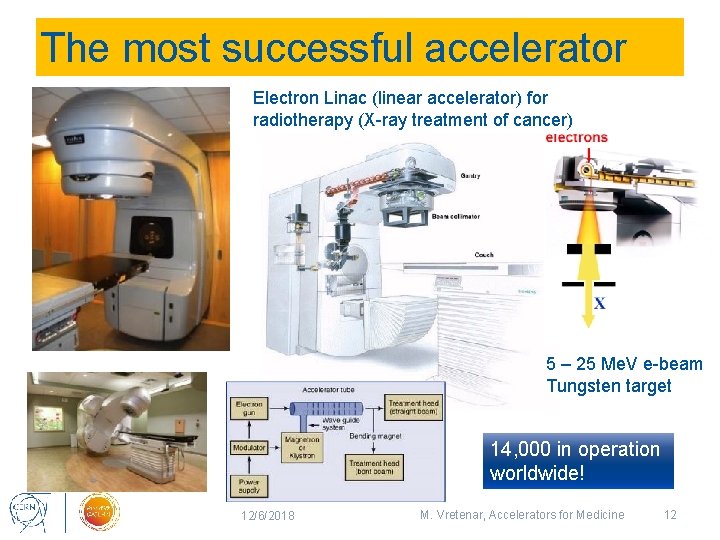
The most successful accelerator Electron Linac (linear accelerator) for radiotherapy (X-ray treatment of cancer) 5 – 25 Me. V e-beam Tungsten target 14, 000 in operation worldwide! 12/6/2018 M. Vretenar, Accelerators for Medicine 12
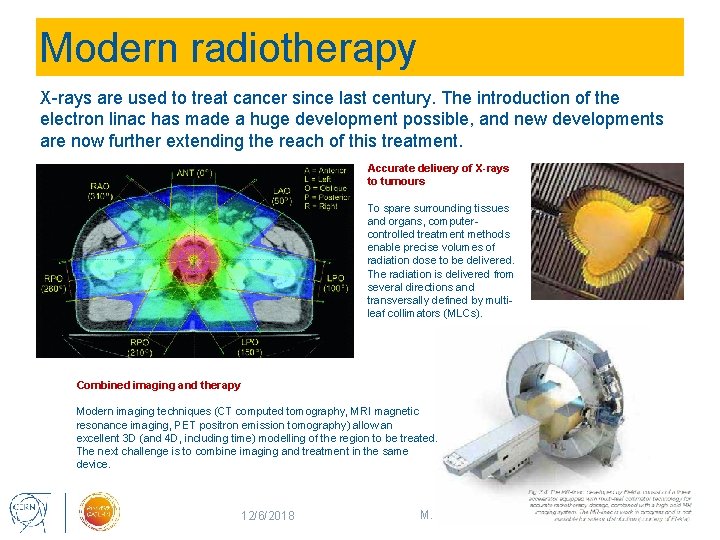
Modern radiotherapy X-rays are used to treat cancer since last century. The introduction of the electron linac has made a huge development possible, and new developments are now further extending the reach of this treatment. Accurate delivery of X-rays to tumours To spare surrounding tissues and organs, computercontrolled treatment methods enable precise volumes of radiation dose to be delivered. The radiation is delivered from several directions and transversally defined by multileaf collimators (MLCs). Combined imaging and therapy Modern imaging techniques (CT computed tomography, MRI magnetic resonance imaging, PET positron emission tomography) allow an excellent 3 D (and 4 D, including time) modelling of the region to be treated. The next challenge is to combine imaging and treatment in the same device. 12/6/2018 M. Vretenar, Accelerators for Medicine 13
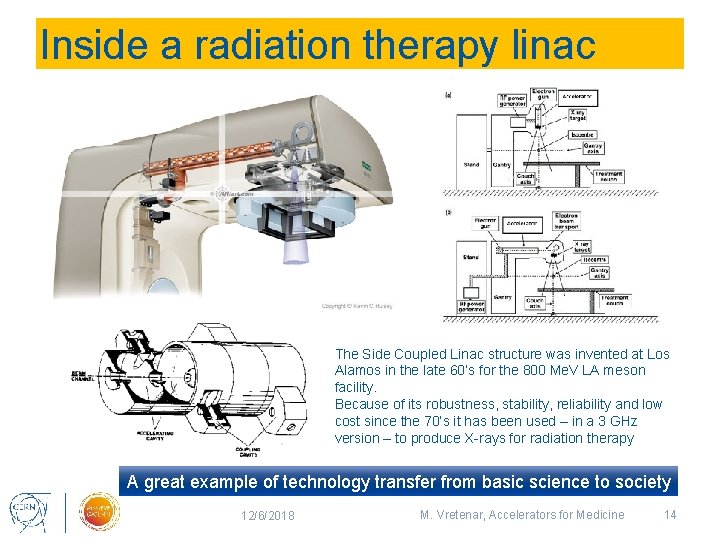
Inside a radiation therapy linac The Side Coupled Linac structure was invented at Los Alamos in the late 60’s for the 800 Me. V LA meson facility. Because of its robustness, stability, reliability and low cost since the 70’s it has been used – in a 3 GHz version – to produce X-rays for radiation therapy A great example of technology transfer from basic science to society 12/6/2018 M. Vretenar, Accelerators for Medicine 14
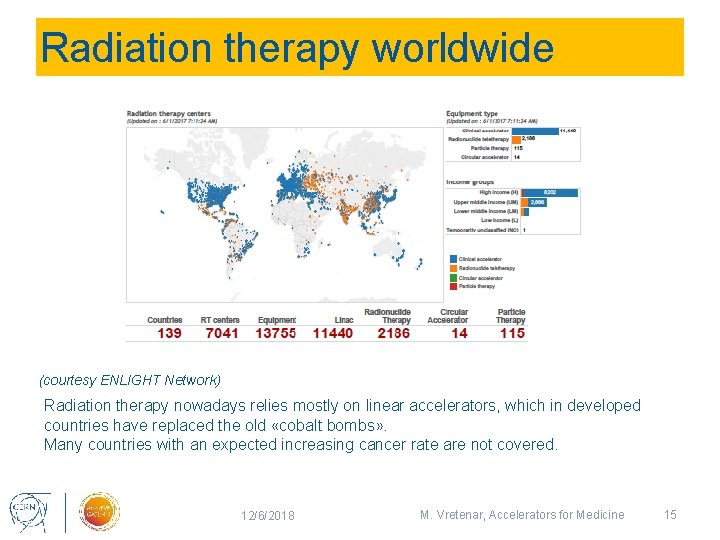
Radiation therapy worldwide (courtesy ENLIGHT Network) Radiation therapy nowadays relies mostly on linear accelerators, which in developed countries have replaced the old «cobalt bombs» . Many countries with an expected increasing cancer rate are not covered. 12/6/2018 M. Vretenar, Accelerators for Medicine 15
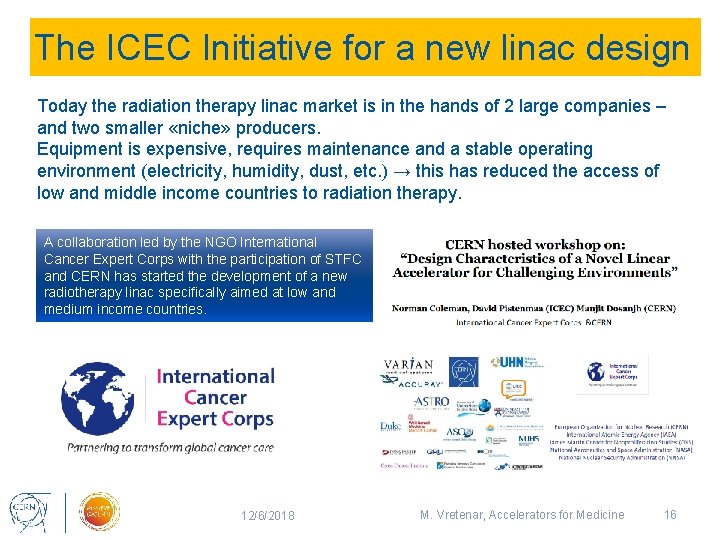
The ICEC Initiative for a new linac design Today the radiation therapy linac market is in the hands of 2 large companies – and two smaller «niche» producers. Equipment is expensive, requires maintenance and a stable operating environment (electricity, humidity, dust, etc. ) → this has reduced the access of low and middle income countries to radiation therapy. A collaboration led by the NGO International Cancer Expert Corps with the participation of STFC and CERN has started the development of a new radiotherapy linac specifically aimed at low and medium income countries. 12/6/2018 M. Vretenar, Accelerators for Medicine 16
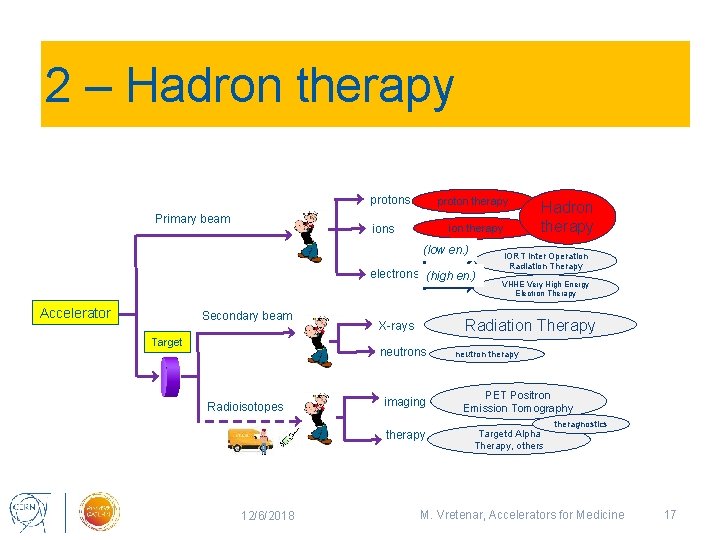
2 – Hadron therapy protons Primary beam proton therapy ions ion therapy (low en. ) electrons (high en. ) Accelerator Secondary beam Target imaging therapy 12/6/2018 IORT Inter Operation Radiation Therapy VHHE Very High Energy Electron Therapy Radiation Therapy X-rays neutrons Radioisotopes Hadron therapy neutron therapy PET Positron Emission Tomography Targetd Alpha Therapy, others theragnostics M. Vretenar, Accelerators for Medicine 17
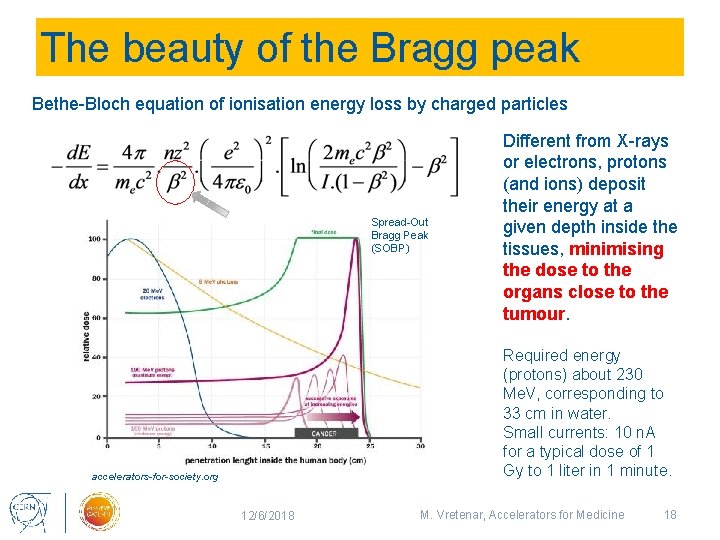
The beauty of the Bragg peak Bethe-Bloch equation of ionisation energy loss by charged particles Spread-Out Bragg Peak (SOBP) Different from X-rays or electrons, protons (and ions) deposit their energy at a given depth inside the tissues, minimising the dose to the organs close to the tumour. Required energy (protons) about 230 Me. V, corresponding to 33 cm in water. Small currents: 10 n. A for a typical dose of 1 Gy to 1 liter in 1 minute. accelerators-for-society. org 12/6/2018 M. Vretenar, Accelerators for Medicine 18
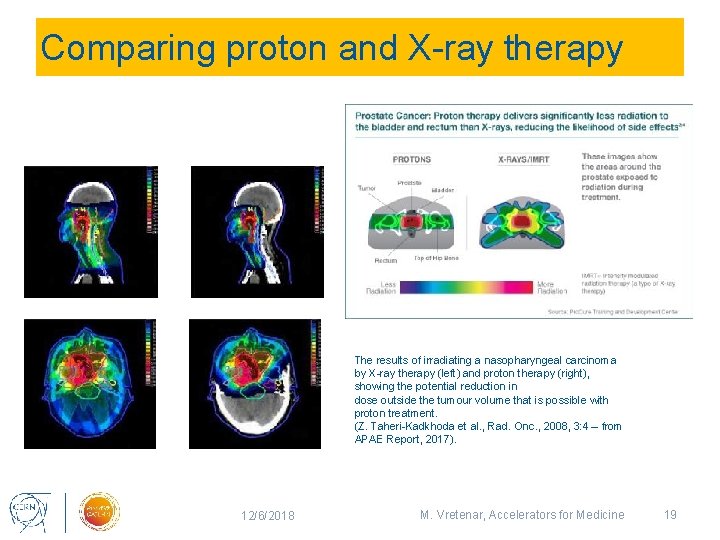
Comparing proton and X-ray therapy The results of irradiating a nasopharyngeal carcinoma by X-ray therapy (left) and proton therapy (right), showing the potential reduction in dose outside the tumour volume that is possible with proton treatment. (Z. Taheri-Kadkhoda et al. , Rad. Onc. , 2008, 3: 4 – from APAE Report, 2017). 12/6/2018 M. Vretenar, Accelerators for Medicine 19
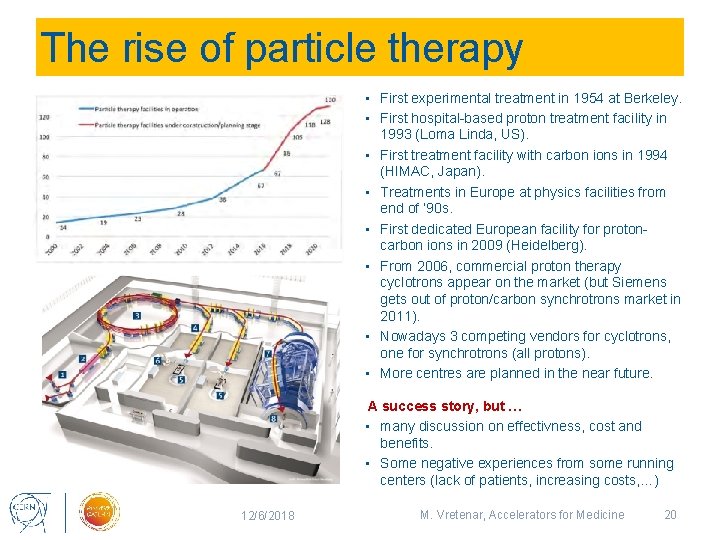
The rise of particle therapy • First experimental treatment in 1954 at Berkeley. • First hospital-based proton treatment facility in • • • 1993 (Loma Linda, US). First treatment facility with carbon ions in 1994 (HIMAC, Japan). Treatments in Europe at physics facilities from end of ‘ 90 s. First dedicated European facility for protoncarbon ions in 2009 (Heidelberg). From 2006, commercial proton therapy cyclotrons appear on the market (but Siemens gets out of proton/carbon synchrotrons market in 2011). Nowadays 3 competing vendors for cyclotrons, one for synchrotrons (all protons). More centres are planned in the near future. A success story, but … • many discussion on effectivness, cost and benefits. • Some negative experiences from some running centers (lack of patients, increasing costs, …) 12/6/2018 M. Vretenar, Accelerators for Medicine 20
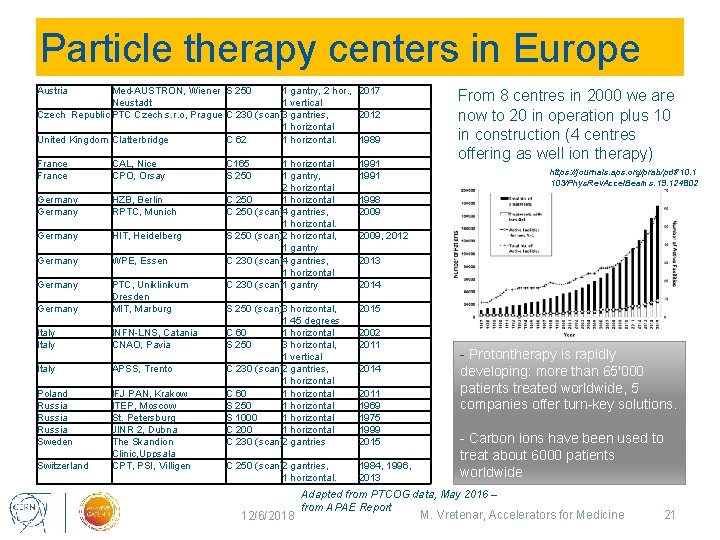
Particle therapy centers in Europe Austria Med-AUSTRON, Wiener S 250 1 gantry, 2 hor. , 2017 Neustadt 1 vertical Czech Republic PTC Czech s. r. o, Prague C 230 (scan)3 gantries, 2012 1 horizontal United Kingdom Clatterbridge C 62 1 horizontal. 1989 France CAL, Nice CPO, Orsay Germany HZB, Berlin RPTC, Munich Germany HIT, Heidelberg Germany WPE, Essen Germany PTC, Uniklinikum Dresden MIT, Marburg Italy INFN-LNS, Catania CNAO, Pavia Italy APSS, Trento Poland Russia Sweden IFJ PAN, Krakow ITEP, Moscow St. Petersburg JINR 2, Dubna The Skandion Clinic, Uppsala CPT, PSI, Villigen Switzerland C 165 S 250 1 horizontal 1 gantry, 2 horizontal C 250 1 horizontal C 250 (scan)4 gantries, 1 horizontal. S 250 (scan)2 horizontal, 1 gantry C 230 (scan)4 gantries, 1 horizontal C 230 (scan)1 gantry 1991 S 250 (scan)3 horizontal, 1 45 degrees C 60 1 horizontal S 250 3 horizontal, 1 vertical C 230 (scan)2 gantries, 1 horizontal C 60 1 horizontal S 250 1 horizontal S 1000 1 horizontal C 230 (scan)2 gantries 2015 C 250 (scan)2 gantries, 1 horizontal. 1984, 1996, 2013 12/6/2018 From 8 centres in 2000 we are now to 20 in operation plus 10 in construction (4 centres offering as well ion therapy) https: //journals. aps. org/prab/pdf/10. 1 103/Phys. Rev. Accel. Beams. 19. 124802 1998 2009, 2012 2013 2014 2002 2011 2014 2011 1969 1975 1999 2015 - Protontherapy is rapidly developing: more than 65'000 patients treated worldwide, 5 companies offer turn-key solutions. - Carbon ions have been used to treat about 6000 patients worldwide Adapted from PTCOG data, May 2016 – from APAE Report M. Vretenar, Accelerators for Medicine 21
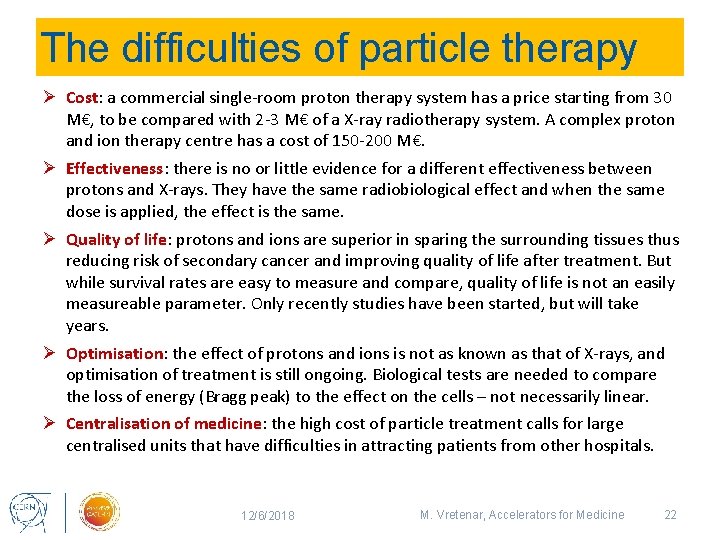
The difficulties of particle therapy Ø Cost: a commercial single-room proton therapy system has a price starting from 30 M€, to be compared with 2 -3 M€ of a X-ray radiotherapy system. A complex proton and ion therapy centre has a cost of 150 -200 M€. Ø Effectiveness: there is no or little evidence for a different effectiveness between protons and X-rays. They have the same radiobiological effect and when the same dose is applied, the effect is the same. Ø Quality of life: protons and ions are superior in sparing the surrounding tissues thus reducing risk of secondary cancer and improving quality of life after treatment. But while survival rates are easy to measure and compare, quality of life is not an easily measureable parameter. Only recently studies have been started, but will take years. Ø Optimisation: the effect of protons and ions is not as known as that of X-rays, and optimisation of treatment is still ongoing. Biological tests are needed to compare the loss of energy (Bragg peak) to the effect on the cells – not necessarily linear. Ø Centralisation of medicine: the high cost of particle treatment calls for large centralised units that have difficulties in attracting patients from other hospitals. 12/6/2018 M. Vretenar, Accelerators for Medicine 22
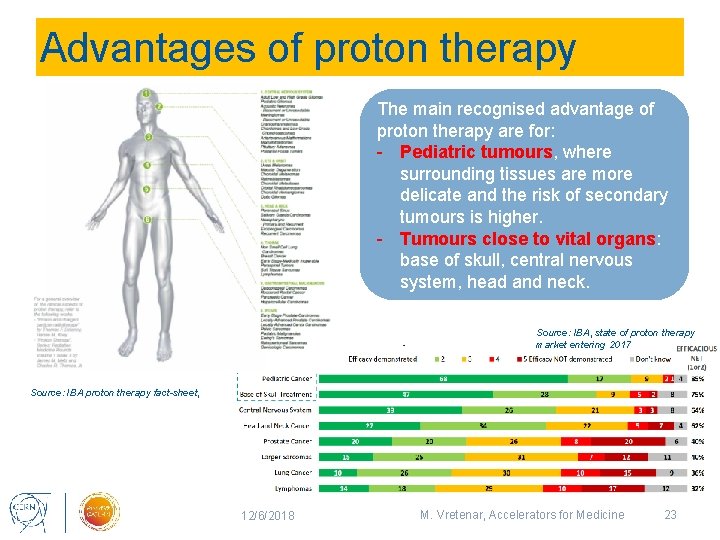
Advantages of proton therapy The main recognised advantage of proton therapy are for: - Pediatric tumours, where surrounding tissues are more delicate and the risk of secondary tumours is higher. - Tumours close to vital organs: base of skull, central nervous system, head and neck. Source: IBA, state of proton therapy market entering 2017 Source: IBA proton therapy fact-sheet, 12/6/2018 M. Vretenar, Accelerators for Medicine 23
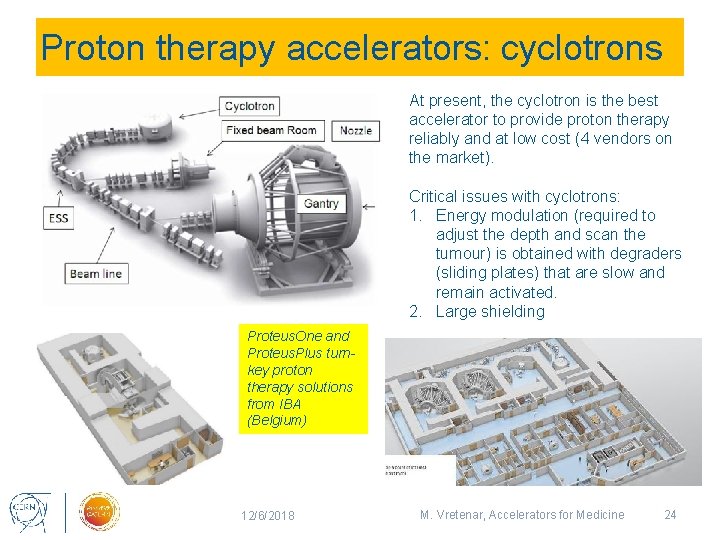
Proton therapy accelerators: cyclotrons At present, the cyclotron is the best accelerator to provide proton therapy reliably and at low cost (4 vendors on the market). Critical issues with cyclotrons: 1. Energy modulation (required to adjust the depth and scan the tumour) is obtained with degraders (sliding plates) that are slow and remain activated. 2. Large shielding Proteus. One and Proteus. Plus turnkey proton therapy solutions from IBA (Belgium) 12/6/2018 M. Vretenar, Accelerators for Medicine 24
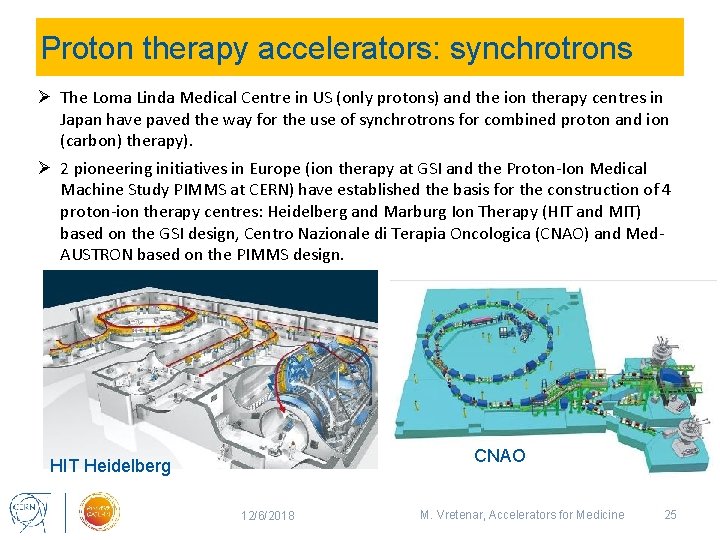
Proton therapy accelerators: synchrotrons Ø The Loma Linda Medical Centre in US (only protons) and the ion therapy centres in Japan have paved the way for the use of synchrotrons for combined proton and ion (carbon) therapy). Ø 2 pioneering initiatives in Europe (ion therapy at GSI and the Proton-Ion Medical Machine Study PIMMS at CERN) have established the basis for the construction of 4 proton-ion therapy centres: Heidelberg and Marburg Ion Therapy (HIT and MIT) based on the GSI design, Centro Nazionale di Terapia Oncologica (CNAO) and Med. AUSTRON based on the PIMMS design. CNAO HIT Heidelberg 12/6/2018 M. Vretenar, Accelerators for Medicine 25
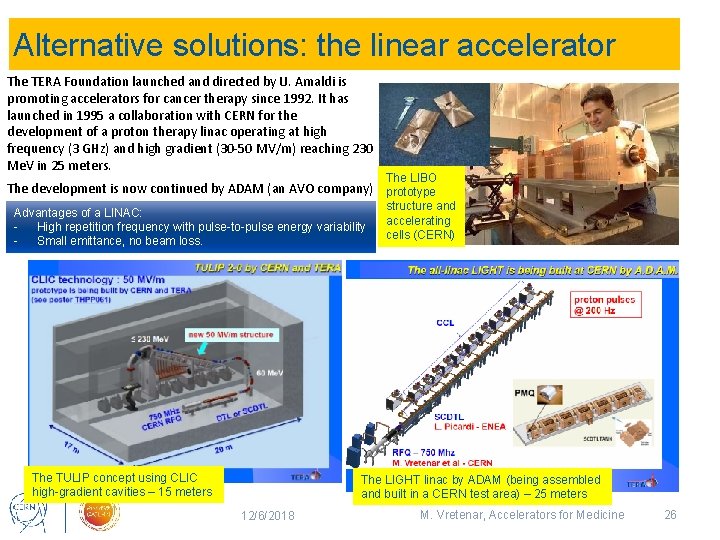
Alternative solutions: the linear accelerator The TERA Foundation launched and directed by U. Amaldi is promoting accelerators for cancer therapy since 1992. It has launched in 1995 a collaboration with CERN for the development of a proton therapy linac operating at high frequency (3 GHz) and high gradient (30 -50 MV/m) reaching 230 Me. V in 25 meters. The LIBO The development is now continued by ADAM (an AVO company) prototype Advantages of a LINAC: - High repetition frequency with pulse-to-pulse energy variability - Small emittance, no beam loss. The TULIP concept using CLIC high-gradient cavities – 15 meters structure and accelerating cells (CERN) The LIGHT linac by ADAM (being assembled and built in a CERN test area) – 25 meters 12/6/2018 M. Vretenar, Accelerators for Medicine 26
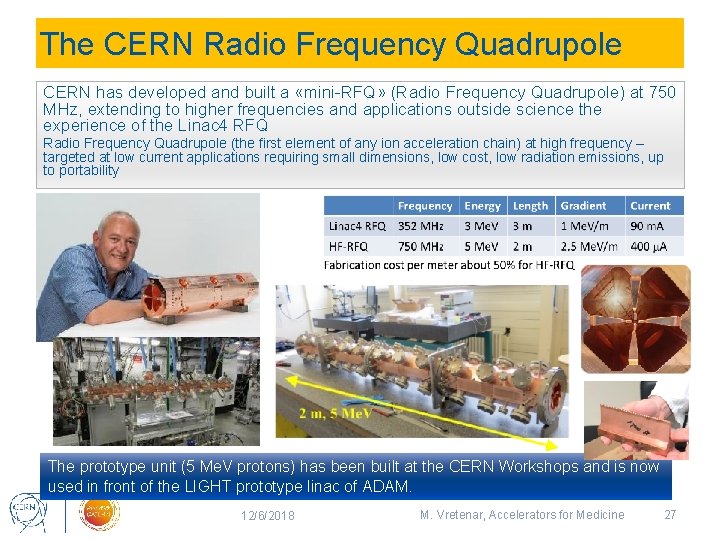
The CERN Radio Frequency Quadrupole CERN has developed and built a «mini-RFQ» (Radio Frequency Quadrupole) at 750 MHz, extending to higher frequencies and applications outside science the experience of the Linac 4 RFQ Radio Frequency Quadrupole (the first element of any ion acceleration chain) at high frequency – targeted at low current applications requiring small dimensions, low cost, low radiation emissions, up to portability The prototype unit (5 Me. V protons) has been built at the CERN Workshops and is now used in front of the LIGHT prototype linac of ADAM. 12/6/2018 M. Vretenar, Accelerators for Medicine 27
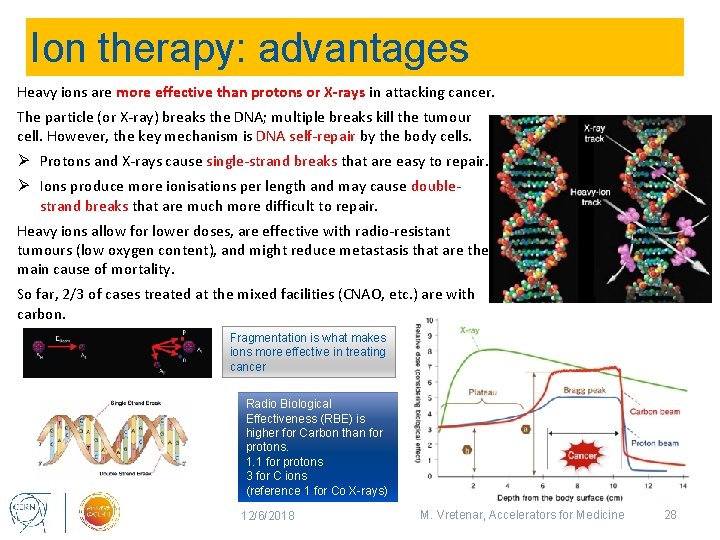
Ion therapy: advantages Heavy ions are more effective than protons or X-rays in attacking cancer. The particle (or X-ray) breaks the DNA; multiple breaks kill the tumour cell. However, the key mechanism is DNA self-repair by the body cells. Ø Protons and X-rays cause single-strand breaks that are easy to repair. Ø Ions produce more ionisations per length and may cause doublestrand breaks that are much more difficult to repair. Heavy ions allow for lower doses, are effective with radio-resistant tumours (low oxygen content), and might reduce metastasis that are the main cause of mortality. So far, 2/3 of cases treated at the mixed facilities (CNAO, etc. ) are with carbon. Fragmentation is what makes ions more effective in treating cancer Radio Biological Effectiveness (RBE) is higher for Carbon than for protons. 1. 1 for protons 3 for C ions (reference 1 for Co X-rays) 12/6/2018 M. Vretenar, Accelerators for Medicine 28
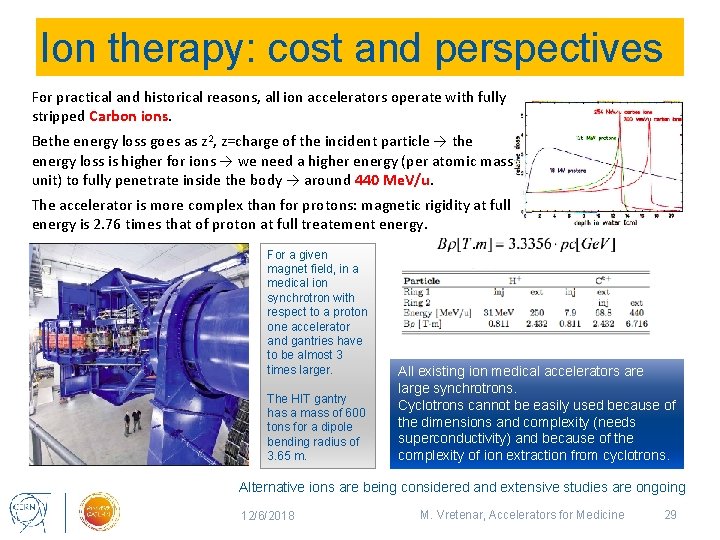
Ion therapy: cost and perspectives For practical and historical reasons, all ion accelerators operate with fully stripped Carbon ions. Bethe energy loss goes as z 2, z=charge of the incident particle → the energy loss is higher for ions → we need a higher energy (per atomic mass unit) to fully penetrate inside the body → around 440 Me. V/u. The accelerator is more complex than for protons: magnetic rigidity at full energy is 2. 76 times that of proton at full treatement energy. For a given magnet field, in a medical ion synchrotron with respect to a proton one accelerator and gantries have to be almost 3 times larger. The HIT gantry has a mass of 600 tons for a dipole bending radius of 3. 65 m. All existing ion medical accelerators are large synchrotrons. Cyclotrons cannot be easily used because of the dimensions and complexity (needs superconductivity) and because of the complexity of ion extraction from cyclotrons. Alternative ions are being considered and extensive studies are ongoing 12/6/2018 M. Vretenar, Accelerators for Medicine 29
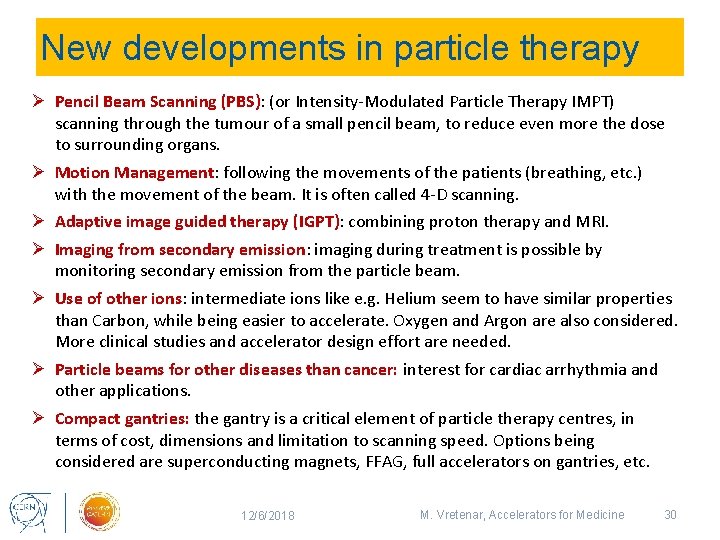
New developments in particle therapy Ø Pencil Beam Scanning (PBS): (or Intensity-Modulated Particle Therapy IMPT) scanning through the tumour of a small pencil beam, to reduce even more the dose to surrounding organs. Ø Motion Management: following the movements of the patients (breathing, etc. ) with the movement of the beam. It is often called 4 -D scanning. Ø Adaptive image guided therapy (IGPT): combining proton therapy and MRI. Ø Imaging from secondary emission: imaging during treatment is possible by monitoring secondary emission from the particle beam. Ø Use of other ions: intermediate ions like e. g. Helium seem to have similar properties than Carbon, while being easier to accelerate. Oxygen and Argon are also considered. More clinical studies and accelerator design effort are needed. Ø Particle beams for other diseases than cancer: interest for cardiac arrhythmia and other applications. Ø Compact gantries: the gantry is a critical element of particle therapy centres, in terms of cost, dimensions and limitation to scanning speed. Options being considered are superconducting magnets, FFAG, full accelerators on gantries, etc. 12/6/2018 M. Vretenar, Accelerators for Medicine 30
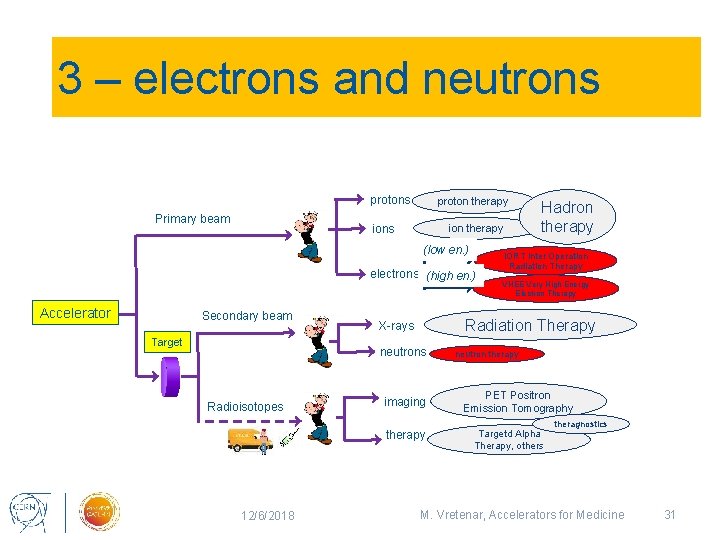
3 – electrons and neutrons protons Primary beam proton therapy ions ion therapy (low en. ) electrons (high en. ) Accelerator Secondary beam Target imaging therapy 12/6/2018 IORT Inter Operation Radiation Therapy VHEE Very High Energy Electron Therapy Radiation Therapy X-rays neutrons Radioisotopes Hadron therapy neutron therapy PET Positron Emission Tomography Targetd Alpha Therapy, others theragnostics M. Vretenar, Accelerators for Medicine 31
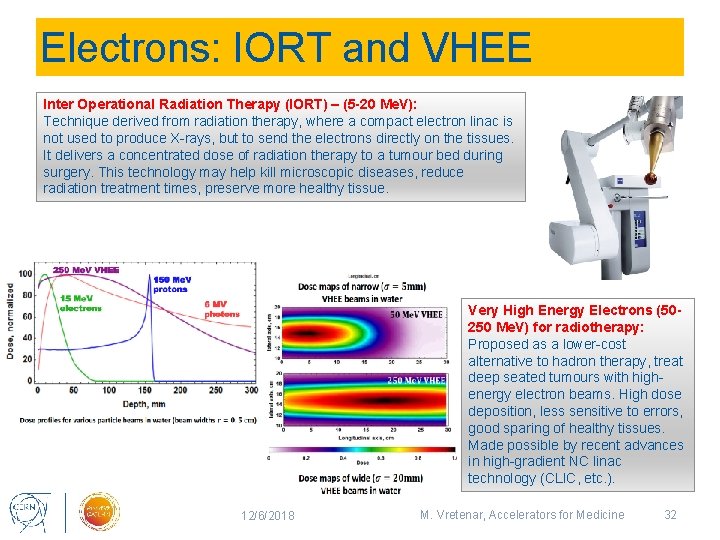
Electrons: IORT and VHEE Inter Operational Radiation Therapy (IORT) – (5 -20 Me. V): Technique derived from radiation therapy, where a compact electron linac is not used to produce X-rays, but to send the electrons directly on the tissues. It delivers a concentrated dose of radiation therapy to a tumour bed during surgery. This technology may help kill microscopic diseases, reduce radiation treatment times, preserve more healthy tissue. Very High Energy Electrons (50250 Me. V) for radiotherapy: Proposed as a lower-cost alternative to hadron therapy, treat deep seated tumours with highenergy electron beams. High dose deposition, less sensitive to errors, good sparing of healthy tissues. Made possible by recent advances in high-gradient NC linac technology (CLIC, etc. ). 12/6/2018 M. Vretenar, Accelerators for Medicine 32
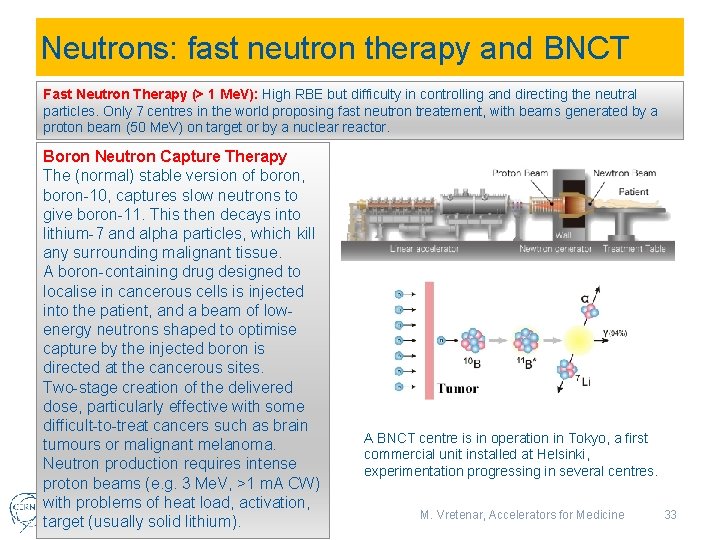
Neutrons: fast neutron therapy and BNCT Fast Neutron Therapy (> 1 Me. V): High RBE but difficulty in controlling and directing the neutral particles. Only 7 centres in the world proposing fast neutron treatement, with beams generated by a proton beam (50 Me. V) on target or by a nuclear reactor. Boron Neutron Capture Therapy The (normal) stable version of boron, boron-10, captures slow neutrons to give boron-11. This then decays into lithium-7 and alpha particles, which kill any surrounding malignant tissue. A boron-containing drug designed to localise in cancerous cells is injected into the patient, and a beam of lowenergy neutrons shaped to optimise capture by the injected boron is directed at the cancerous sites. Two-stage creation of the delivered dose, particularly effective with some difficult-to-treat cancers such as brain tumours or malignant melanoma. Neutron production requires intense proton beams (e. g. 3 Me. V, >1 m. A CW) with problems of heat load, activation, target (usually solid lithium). 12/6/2018 A BNCT centre is in operation in Tokyo, a first commercial unit installed at Helsinki, experimentation progressing in several centres. M. Vretenar, Accelerators for Medicine 33
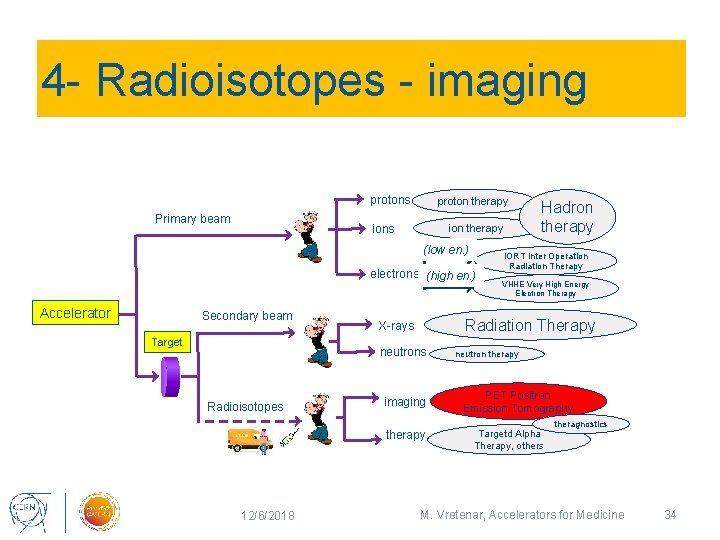
4 - Radioisotopes - imaging protons Primary beam proton therapy ions ion therapy (low en. ) electrons (high en. ) Accelerator Secondary beam Target imaging therapy 12/6/2018 IORT Inter Operation Radiation Therapy VHHE Very High Energy Electron Therapy Radiation Therapy X-rays neutrons Radioisotopes Hadron therapy neutron therapy PET Positron Emission Tomography Targetd Alpha Therapy, others theragnostics M. Vretenar, Accelerators for Medicine 34
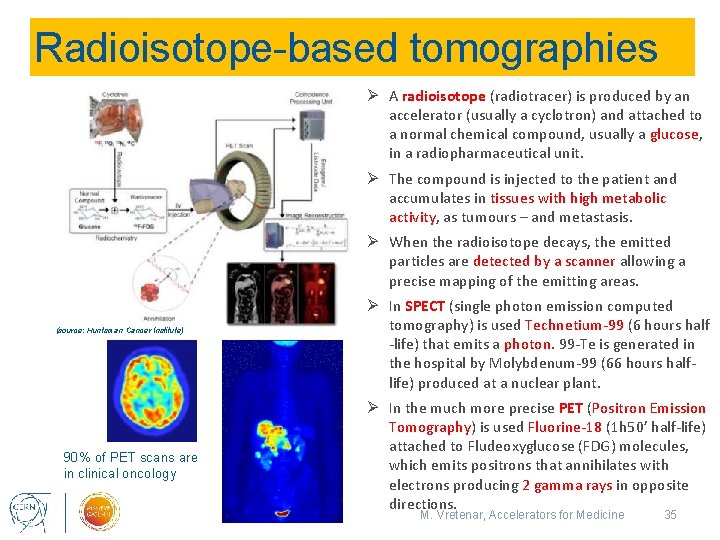
Radioisotope-based tomographies Ø A radioisotope (radiotracer) is produced by an accelerator (usually a cyclotron) and attached to a normal chemical compound, usually a glucose, in a radiopharmaceutical unit. Ø The compound is injected to the patient and accumulates in tissues with high metabolic activity, as tumours – and metastasis. Ø When the radioisotope decays, the emitted particles are detected by a scanner allowing a precise mapping of the emitting areas. Ø In SPECT (single photon emission computed tomography) is used Technetium-99 (6 hours half -life) that emits a photon. 99 -Te is generated in the hospital by Molybdenum-99 (66 hours halflife) produced at a nuclear plant. (source: Huntsman Cancer Institute) 90% of PET scans are in clinical oncology 12/6/2018 Ø In the much more precise PET (Positron Emission Tomography) is used Fluorine-18 (1 h 50’ half-life) attached to Fludeoxyglucose (FDG) molecules, which emits positrons that annihilates with electrons producing 2 gamma rays in opposite directions. M. Vretenar, Accelerators for Medicine 35
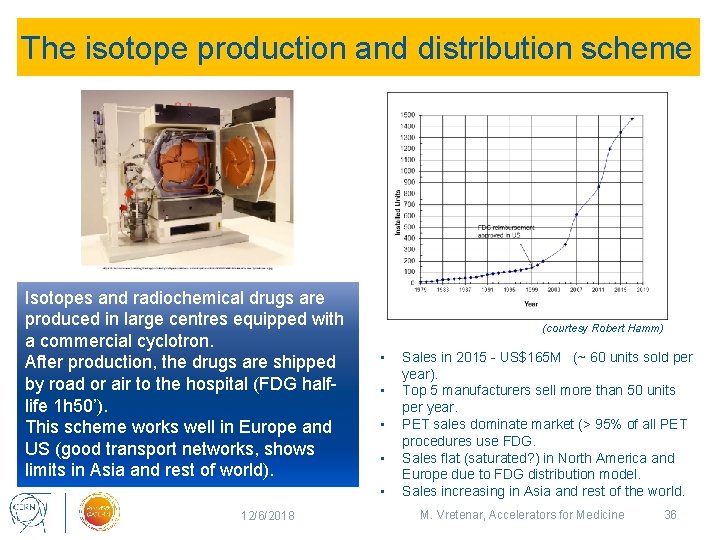
The isotope production and distribution scheme Isotopes and radiochemical drugs are produced in large centres equipped with a commercial cyclotron. After production, the drugs are shipped by road or air to the hospital (FDG halflife 1 h 50’). This scheme works well in Europe and US (good transport networks, shows limits in Asia and rest of world). (courtesy Robert Hamm) • • • 12/6/2018 Sales in 2015 - US$165 M (~ 60 units sold per year). Top 5 manufacturers sell more than 50 units per year. PET sales dominate market (> 95% of all PET procedures use FDG. Sales flat (saturated? ) in North America and Europe due to FDG distribution model. Sales increasing in Asia and rest of the world. M. Vretenar, Accelerators for Medicine 36
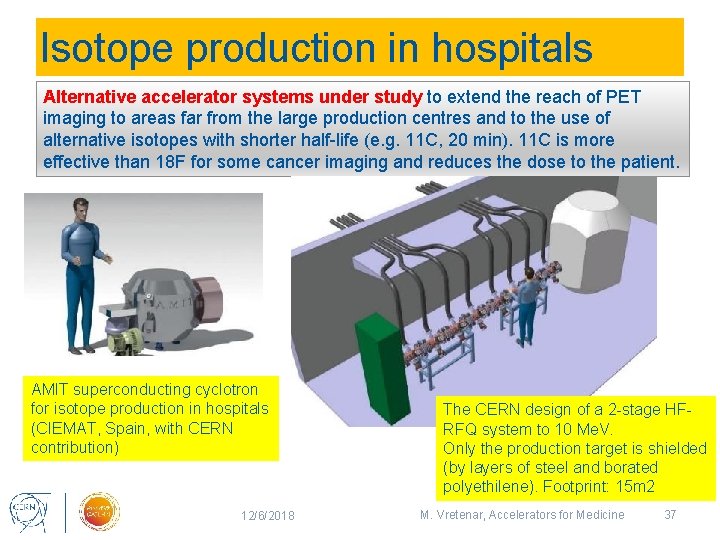
Isotope production in hospitals Alternative accelerator systems under study to extend the reach of PET imaging to areas far from the large production centres and to the use of alternative isotopes with shorter half-life (e. g. 11 C, 20 min). 11 C is more effective than 18 F for some cancer imaging and reduces the dose to the patient. AMIT superconducting cyclotron for isotope production in hospitals (CIEMAT, Spain, with CERN contribution) 12/6/2018 The CERN design of a 2 -stage HFRFQ system to 10 Me. V. Only the production target is shielded (by layers of steel and borated polyethilene). Footprint: 15 m 2 M. Vretenar, Accelerators for Medicine 37
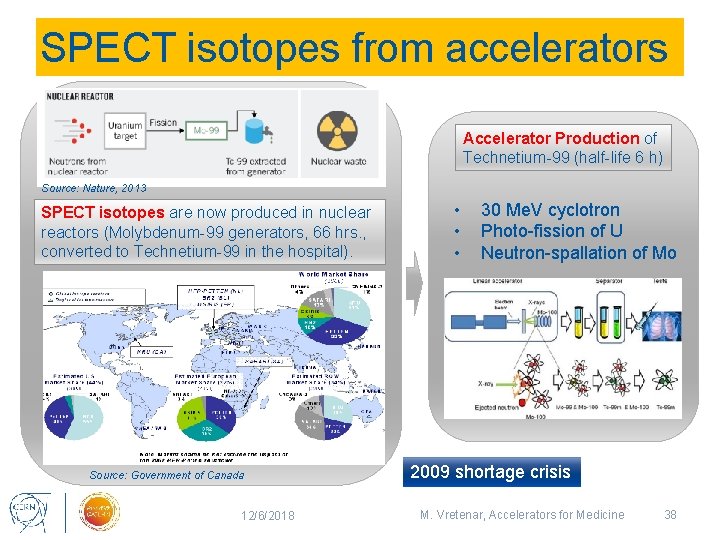
SPECT isotopes from accelerators Accelerator Production of Technetium-99 (half-life 6 h) Source: Nature, 2013 SPECT isotopes are now produced in nuclear reactors (Molybdenum-99 generators, 66 hrs. , converted to Technetium-99 in the hospital). Source: Government of Canada 12/6/2018 • • • 30 Me. V cyclotron Photo-fission of U Neutron-spallation of Mo 2009 shortage crisis M. Vretenar, Accelerators for Medicine 38
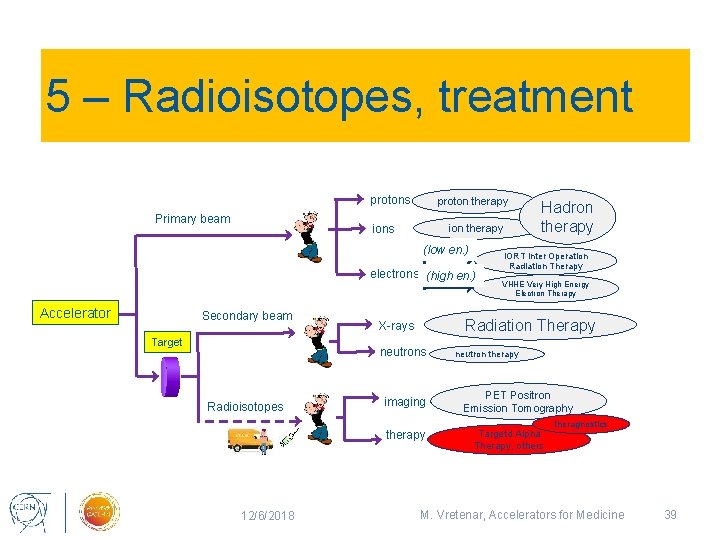
5 – Radioisotopes, treatment protons Primary beam proton therapy ions ion therapy (low en. ) electrons (high en. ) Accelerator Secondary beam Target imaging therapy 12/6/2018 IORT Inter Operation Radiation Therapy VHHE Very High Energy Electron Therapy Radiation Therapy X-rays neutrons Radioisotopes Hadron therapy neutron therapy PET Positron Emission Tomography Targetd Alpha Therapy, others theragnostics M. Vretenar, Accelerators for Medicine 39
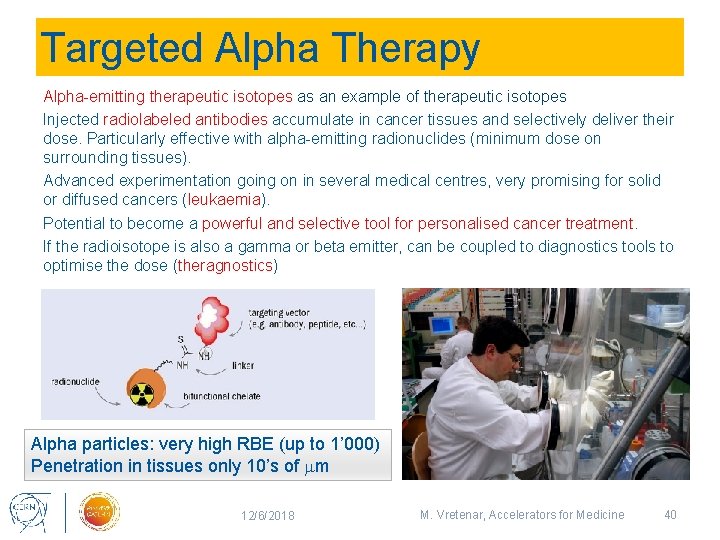
Targeted Alpha Therapy Alpha-emitting therapeutic isotopes as an example of therapeutic isotopes Injected radiolabeled antibodies accumulate in cancer tissues and selectively deliver their dose. Particularly effective with alpha-emitting radionuclides (minimum dose on surrounding tissues). Advanced experimentation going on in several medical centres, very promising for solid or diffused cancers (leukaemia). Potential to become a powerful and selective tool for personalised cancer treatment. If the radioisotope is also a gamma or beta emitter, can be coupled to diagnostics tools to optimise the dose (theragnostics) Alpha particles: very high RBE (up to 1’ 000) Penetration in tissues only 10’s of mm 12/6/2018 M. Vretenar, Accelerators for Medicine 40
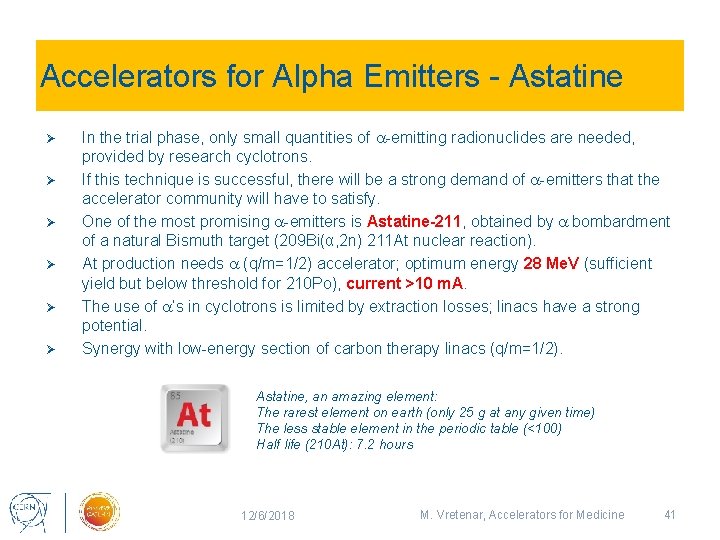
Accelerators for Alpha Emitters - Astatine Ø Ø Ø In the trial phase, only small quantities of a-emitting radionuclides are needed, provided by research cyclotrons. If this technique is successful, there will be a strong demand of a-emitters that the accelerator community will have to satisfy. One of the most promising a-emitters is Astatine-211, obtained by a bombardment of a natural Bismuth target (209 Bi(α, 2 n) 211 At nuclear reaction). At production needs a (q/m=1/2) accelerator; optimum energy 28 Me. V (sufficient yield but below threshold for 210 Po), current >10 m. A. The use of a’s in cyclotrons is limited by extraction losses; linacs have a strong potential. Synergy with low-energy section of carbon therapy linacs (q/m=1/2). Astatine, an amazing element: The rarest element on earth (only 25 g at any given time) The less stable element in the periodic table (<100) Half life (210 At): 7. 2 hours 12/6/2018 M. Vretenar, Accelerators for Medicine 41
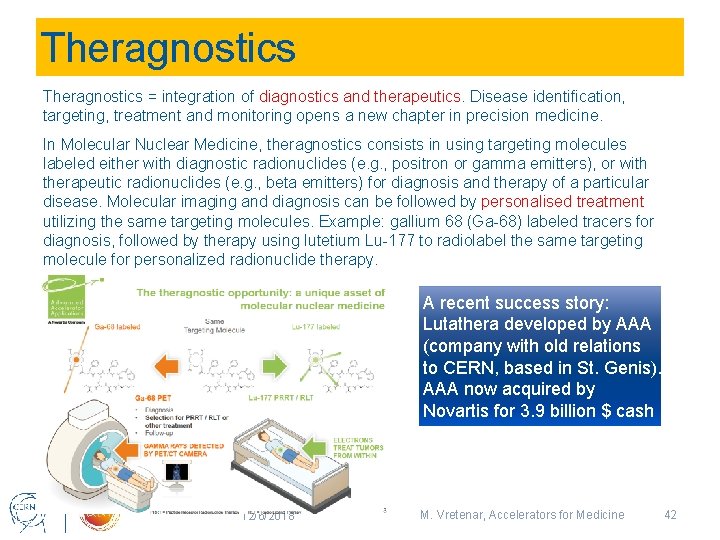
Theragnostics = integration of diagnostics and therapeutics. Disease identification, targeting, treatment and monitoring opens a new chapter in precision medicine. In Molecular Nuclear Medicine, theragnostics consists in using targeting molecules labeled either with diagnostic radionuclides (e. g. , positron or gamma emitters), or with therapeutic radionuclides (e. g. , beta emitters) for diagnosis and therapy of a particular disease. Molecular imaging and diagnosis can be followed by personalised treatment utilizing the same targeting molecules. Example: gallium 68 (Ga-68) labeled tracers for diagnosis, followed by therapy using lutetium Lu-177 to radiolabel the same targeting molecule for personalized radionuclide therapy. A recent success story: Lutathera developed by AAA (company with old relations to CERN, based in St. Genis). AAA now acquired by Novartis for 3. 9 billion $ cash 12/6/2018 M. Vretenar, Accelerators for Medicine 42
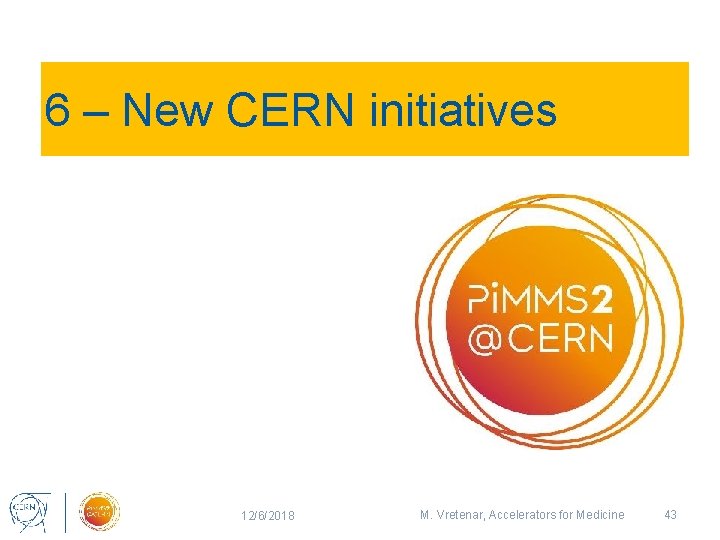
6 – New CERN initiatives 12/6/2018 M. Vretenar, Accelerators for Medicine 43
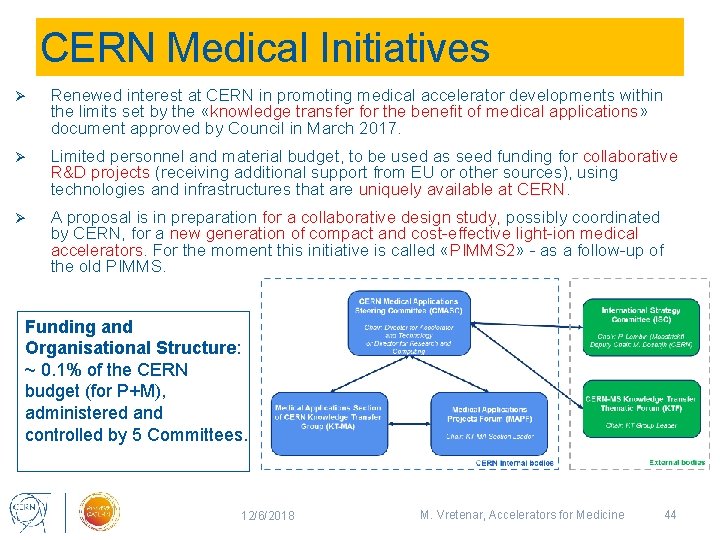
CERN Medical Initiatives Ø Renewed interest at CERN in promoting medical accelerator developments within the limits set by the «knowledge transfer for the benefit of medical applications» document approved by Council in March 2017. Ø Limited personnel and material budget, to be used as seed funding for collaborative R&D projects (receiving additional support from EU or other sources), using technologies and infrastructures that are uniquely available at CERN. Ø A proposal is in preparation for a collaborative design study, possibly coordinated by CERN, for a new generation of compact and cost-effective light-ion medical accelerators. For the moment this initiative is called «PIMMS 2» - as a follow-up of the old PIMMS. Funding and Organisational Structure: ~ 0. 1% of the CERN budget (for P+M), administered and controlled by 5 Committees. 12/6/2018 M. Vretenar, Accelerators for Medicine 44
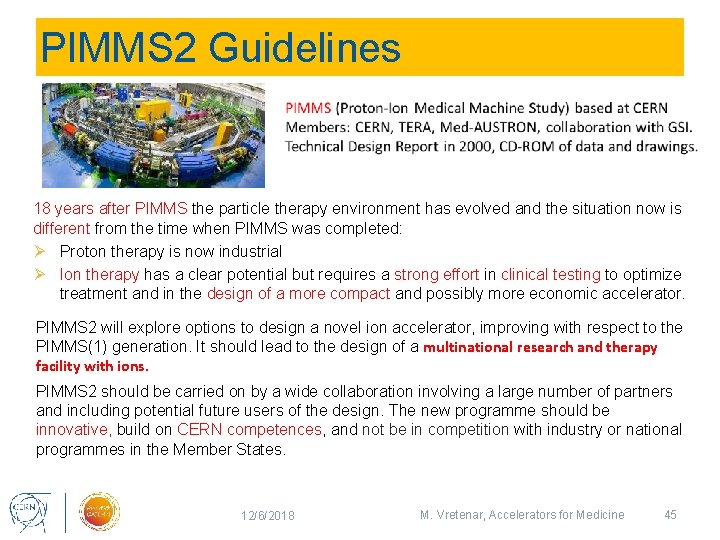
PIMMS 2 Guidelines 18 years after PIMMS the particle therapy environment has evolved and the situation now is different from the time when PIMMS was completed: Ø Proton therapy is now industrial Ø Ion therapy has a clear potential but requires a strong effort in clinical testing to optimize treatment and in the design of a more compact and possibly more economic accelerator. PIMMS 2 will explore options to design a novel ion accelerator, improving with respect to the PIMMS(1) generation. It should lead to the design of a multinational research and therapy facility with ions. PIMMS 2 should be carried on by a wide collaboration involving a large number of partners and including potential future users of the design. The new programme should be innovative, build on CERN competences, and not be in competition with industry or national programmes in the Member States. 12/6/2018 M. Vretenar, Accelerators for Medicine 45
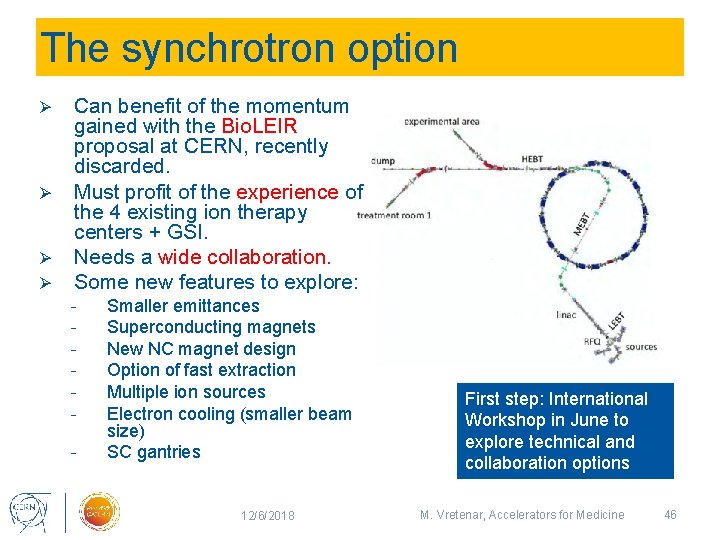
The synchrotron option Ø Ø Can benefit of the momentum gained with the Bio. LEIR proposal at CERN, recently discarded. Must profit of the experience of the 4 existing ion therapy centers + GSI. Needs a wide collaboration. Some new features to explore: - Smaller emittances Superconducting magnets New NC magnet design Option of fast extraction Multiple ion sources Electron cooling (smaller beam size) SC gantries 12/6/2018 First step: International Workshop in June to explore technical and collaboration options M. Vretenar, Accelerators for Medicine 46
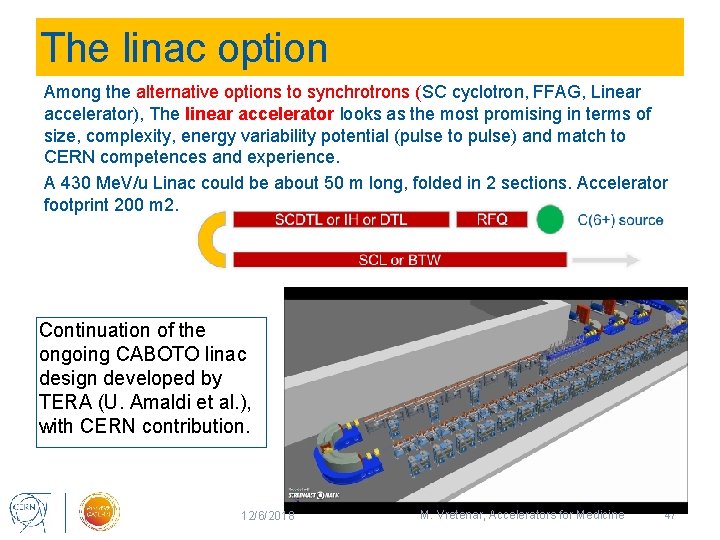
The linac option Among the alternative options to synchrotrons (SC cyclotron, FFAG, Linear accelerator), The linear accelerator looks as the most promising in terms of size, complexity, energy variability potential (pulse to pulse) and match to CERN competences and experience. A 430 Me. V/u Linac could be about 50 m long, folded in 2 sections. Accelerator footprint 200 m 2. Continuation of the ongoing CABOTO linac design developed by TERA (U. Amaldi et al. ), with CERN contribution. 12/6/2018 M. Vretenar, Accelerators for Medicine 47
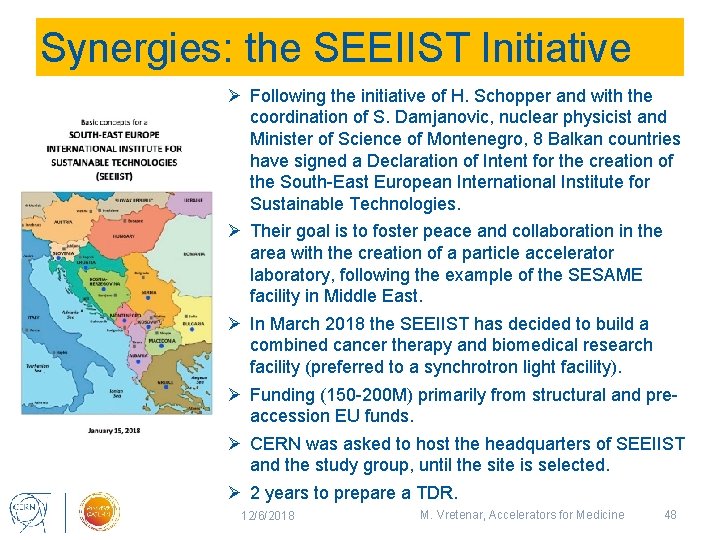
Synergies: the SEEIIST Initiative Ø Following the initiative of H. Schopper and with the coordination of S. Damjanovic, nuclear physicist and Minister of Science of Montenegro, 8 Balkan countries have signed a Declaration of Intent for the creation of the South-East European International Institute for Sustainable Technologies. Ø Their goal is to foster peace and collaboration in the area with the creation of a particle accelerator laboratory, following the example of the SESAME facility in Middle East. Ø In March 2018 the SEEIIST has decided to build a combined cancer therapy and biomedical research facility (preferred to a synchrotron light facility). Ø Funding (150 -200 M) primarily from structural and preaccession EU funds. Ø CERN was asked to host the headquarters of SEEIIST and the study group, until the site is selected. Ø 2 years to prepare a TDR. 12/6/2018 M. Vretenar, Accelerators for Medicine 48
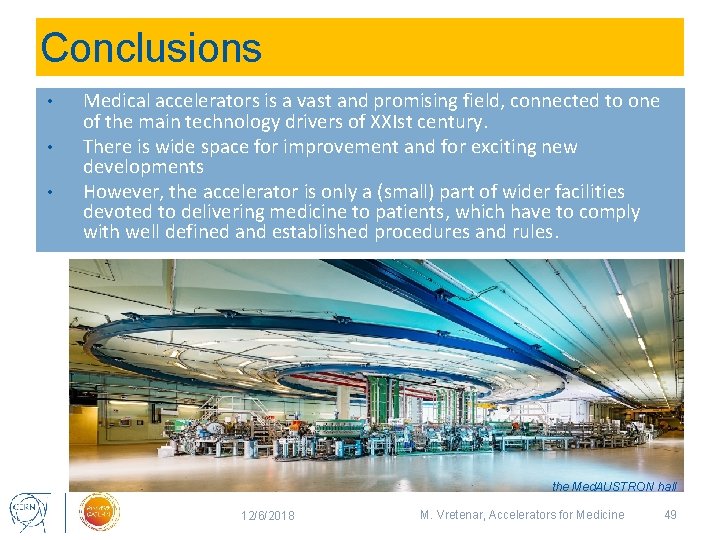
Conclusions • • • Medical accelerators is a vast and promising field, connected to one of the main technology drivers of XXIst century. There is wide space for improvement and for exciting new developments However, the accelerator is only a (small) part of wider facilities devoted to delivering medicine to patients, which have to comply with well defined and established procedures and rules. the Med. AUSTRON hall 12/6/2018 M. Vretenar, Accelerators for Medicine 49
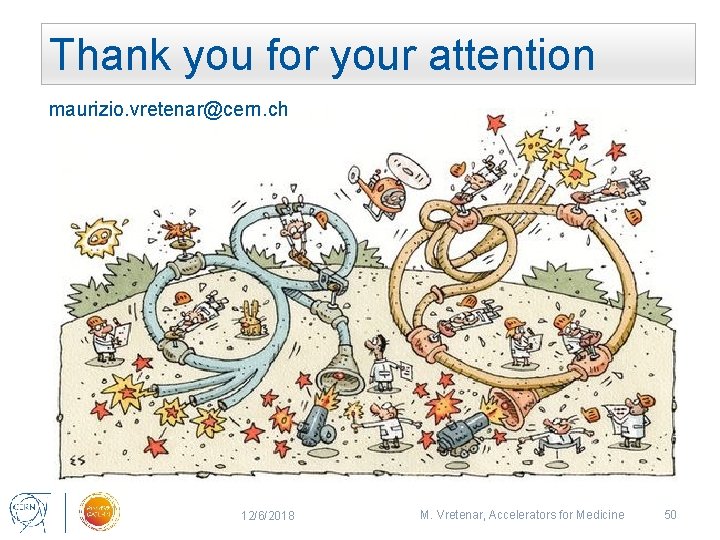
Thank you for your attention maurizio. vretenar@cern. ch 12/6/2018 M. Vretenar, Accelerators for Medicine 50
Cern
Maurizio vretenar
Maurizio vretenar
Good to great technology accelerators
The long-term future of particle accelerators
Good to great chapter 7 summary
Accelerators computer architecture
Cosmic super accelerators
Slidetodoc
Set current query acceleration
Cern academic training
Academic medicine
Maurizio allegri
Maurizio bottoni pittore
Maurizio
Maria grazia ortoleva
Maurizio cattelan
Dr maurizio cecchini
Avv maurizio cenci
Marte di todi
Maurizio fauri
Maurizio
Maurizio paci
Maurizio sciglio
Maurizio muraglia
Dermatologo nuova ricerca rimini
Maurizio trevisani
Maurizio
Maurizio carrino cardarelli
Flogogen
Associazione aicap
Sebastiano maurizio messina
Aragrande
Maurizio grilli
Maurizio salaris
Geometri för barn
Bästa kameran för astrofoto
Mat för idrottare
Etik och ledarskap etisk kod för chefer
Publik sektor
Personlig tidbok för yrkesförare
Datorkunskap för nybörjare
Klädsel i rom
Kontinuitetshantering
Vilken grundregel finns det för tronföljden i sverige?
Rita perspektiv
Ministerstyre för och nackdelar
Jätte råtta
Kanaans land
Sju principer för tillitsbaserad styrning
Nyckelkompetenser för livslångt lärande