Transport Processes Overall heat transfer coefficient From previous
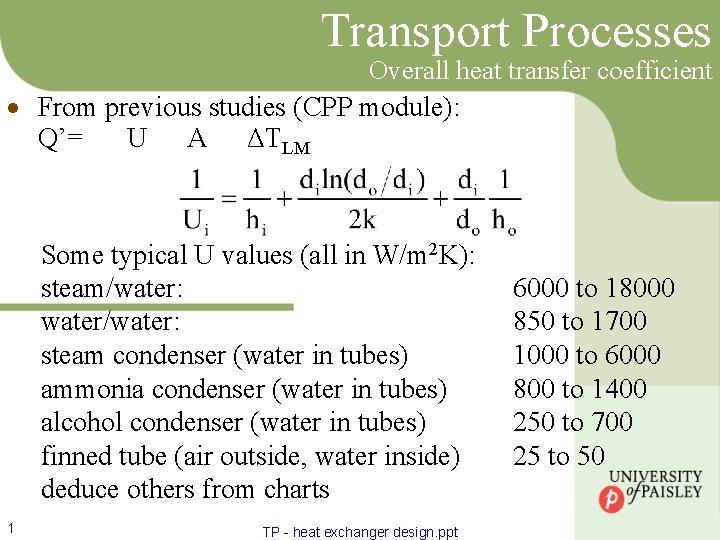
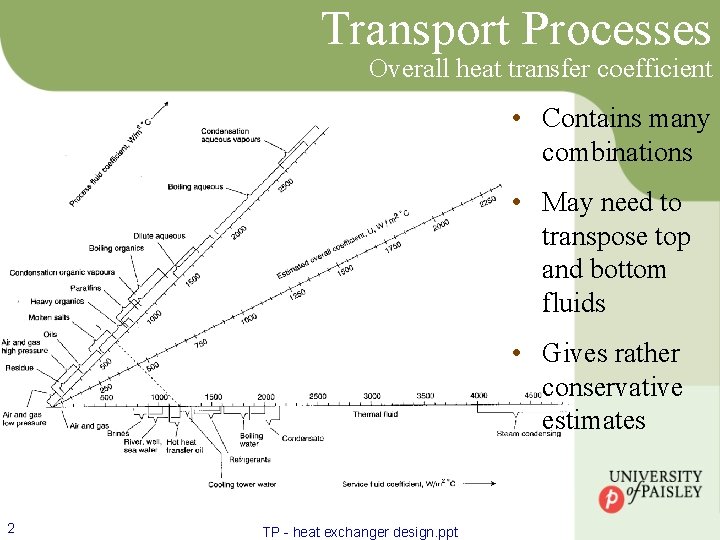
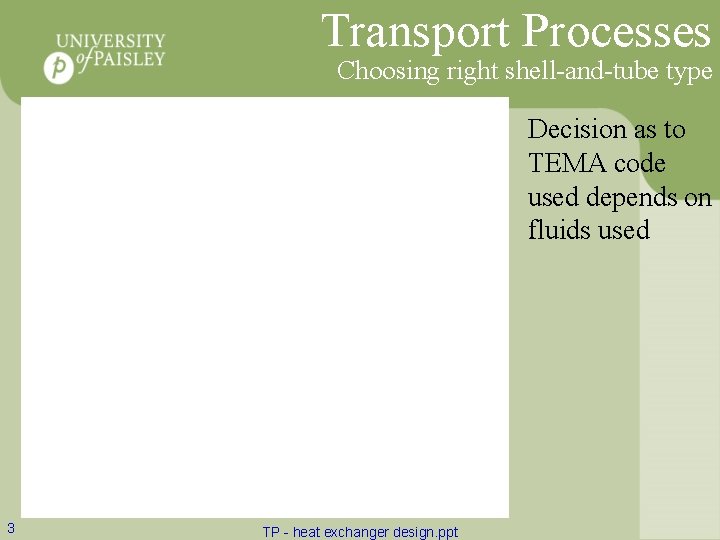
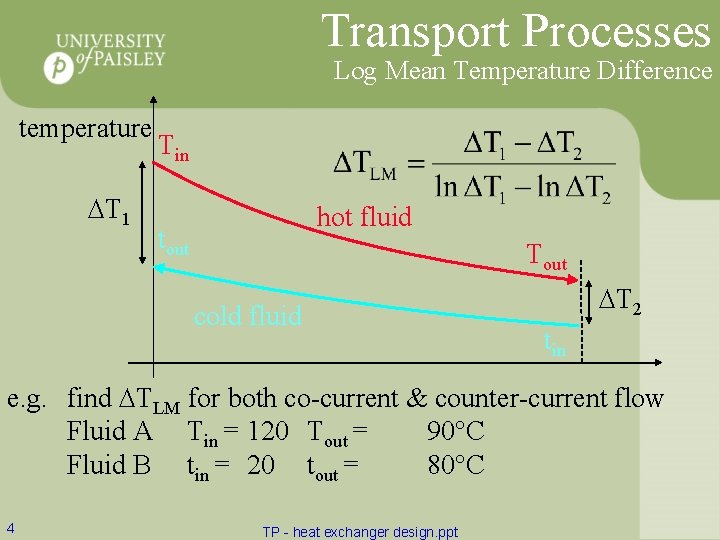
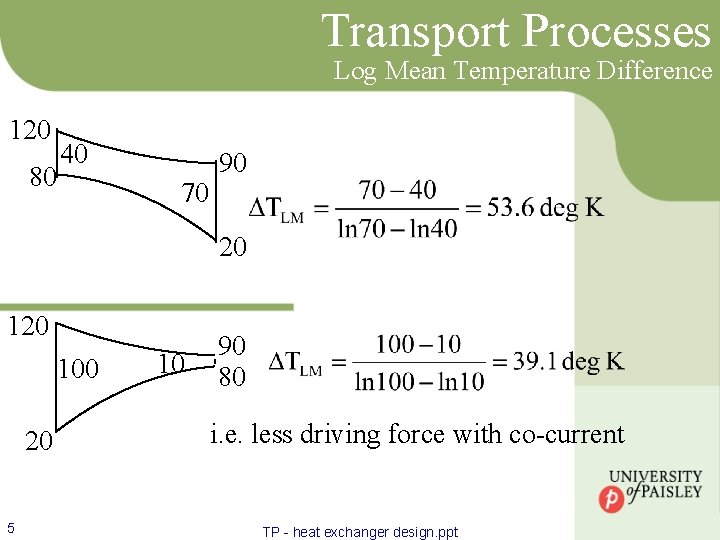
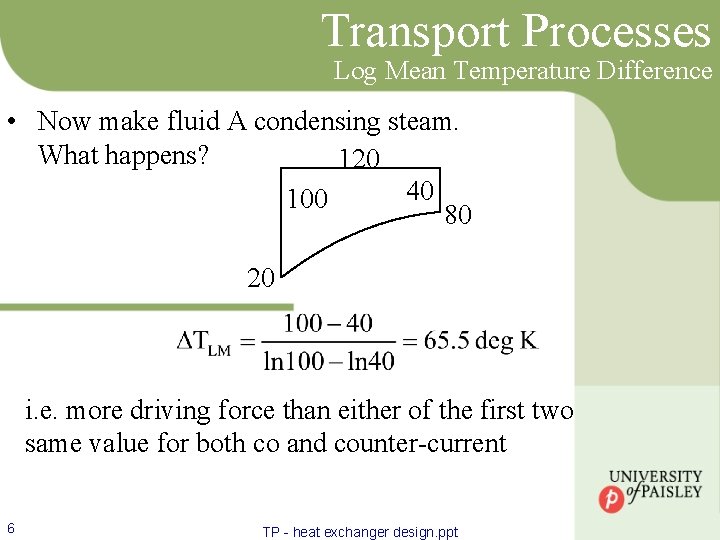
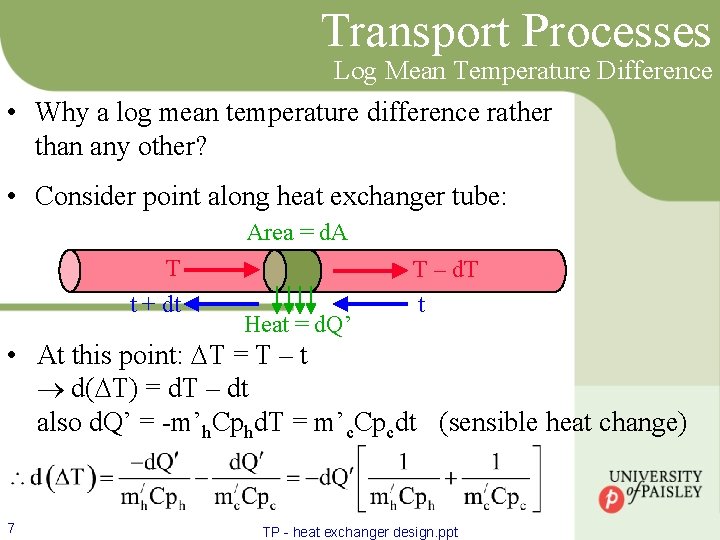
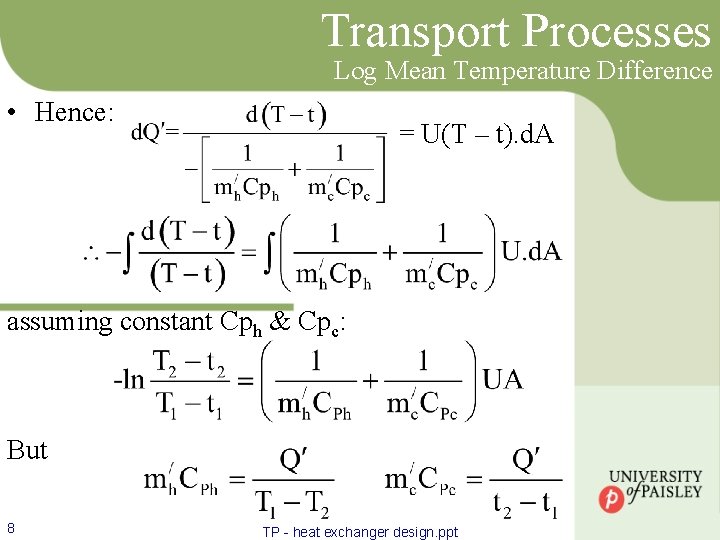
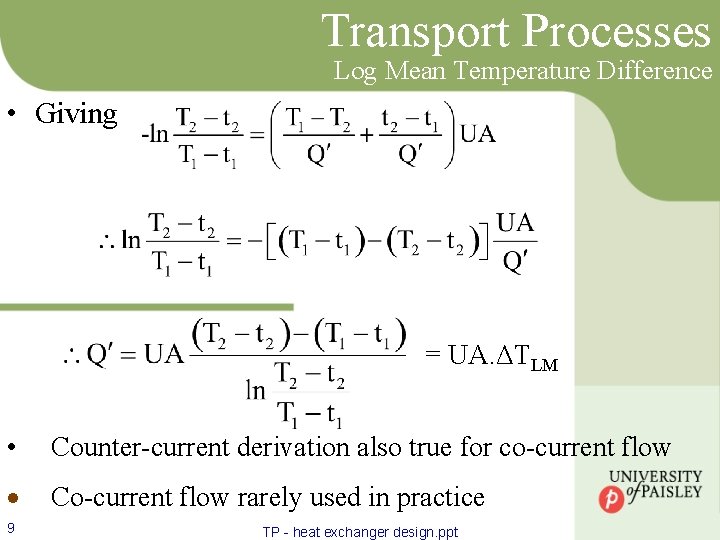
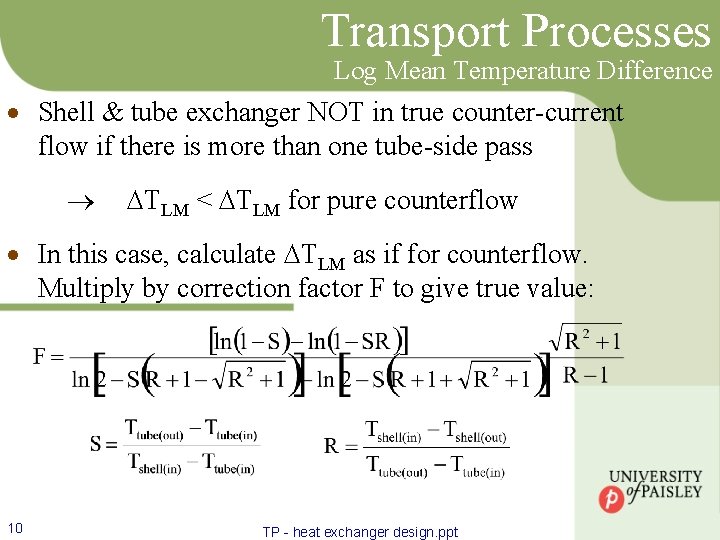
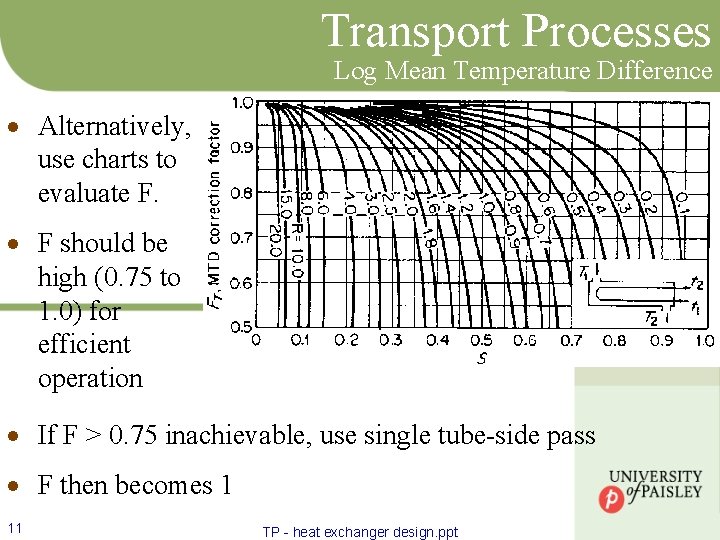
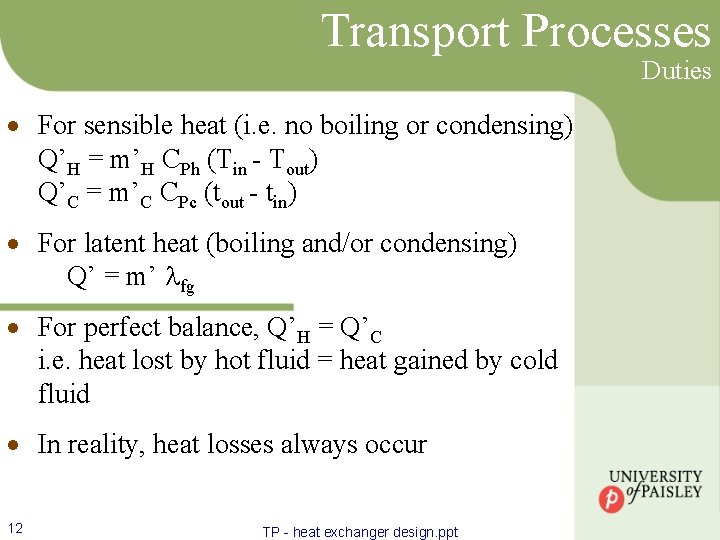
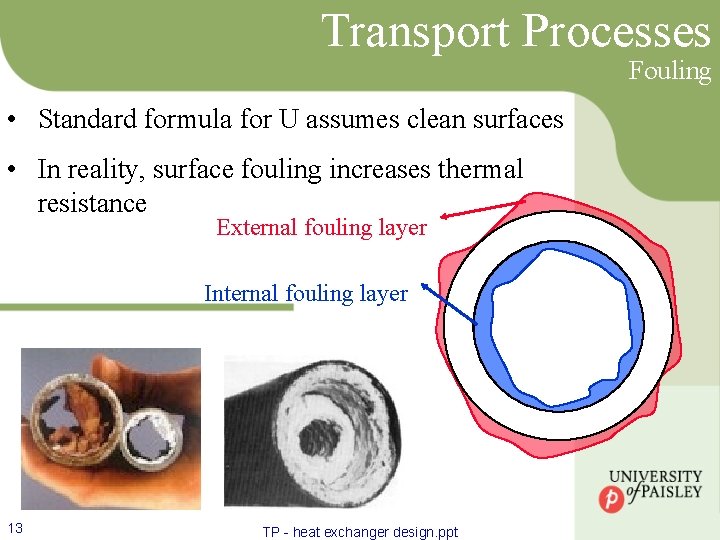
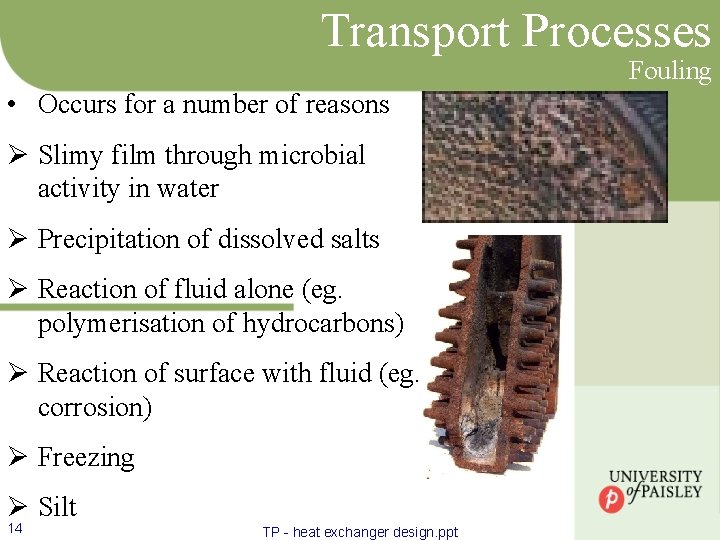
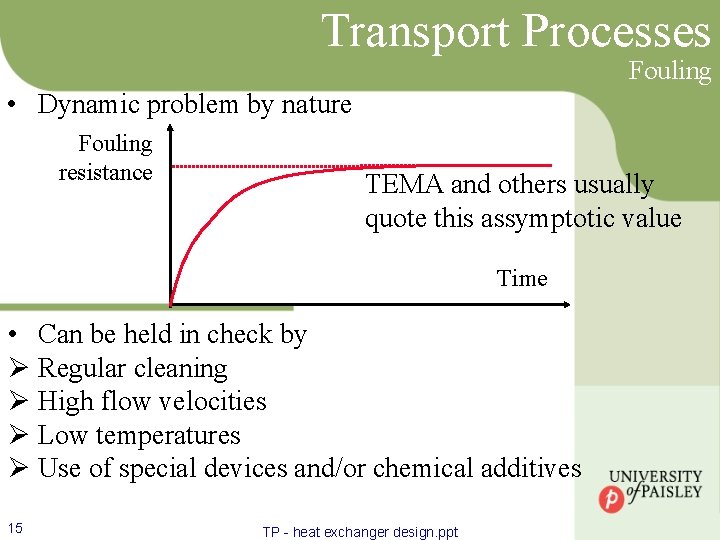
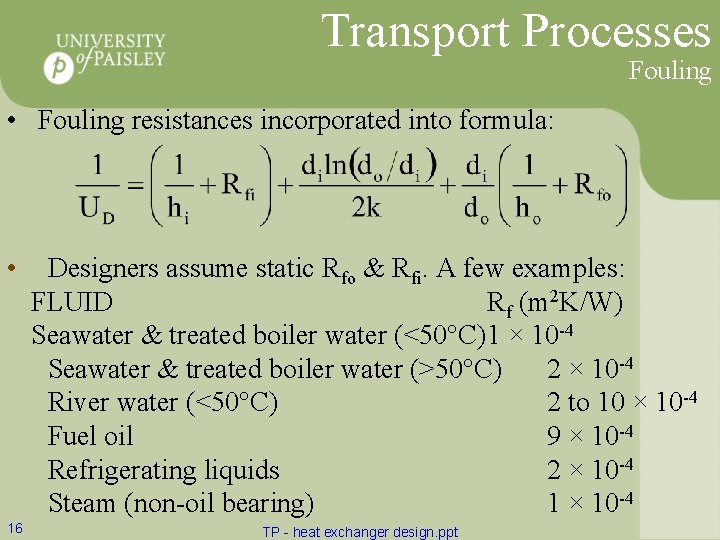
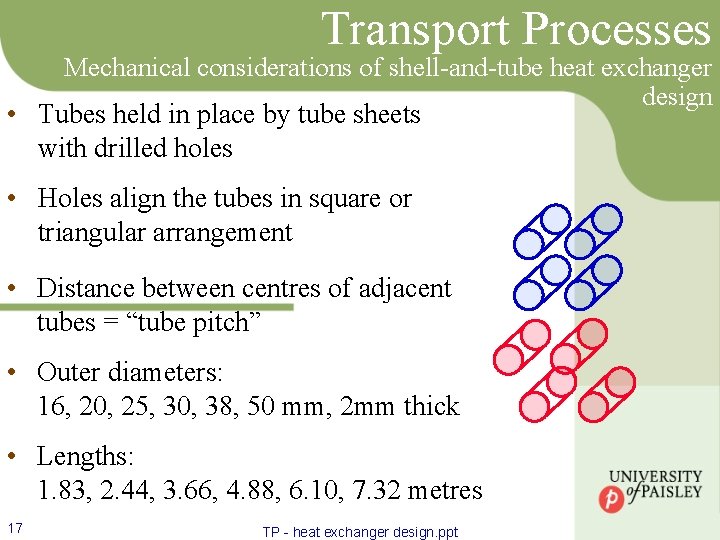
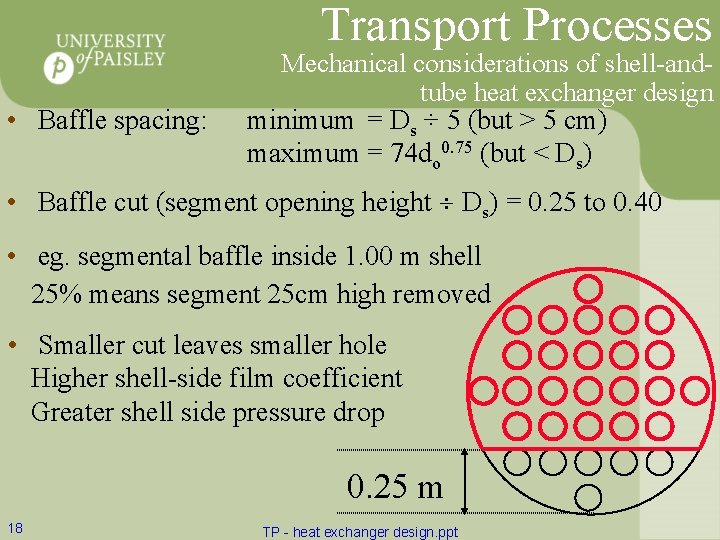
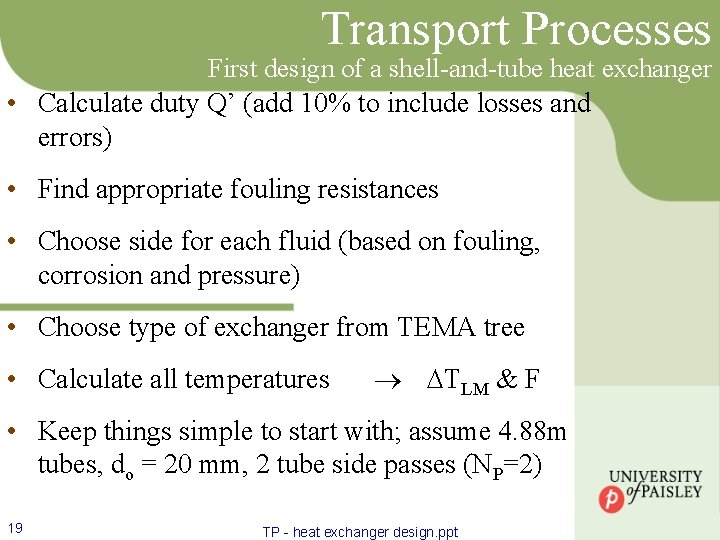
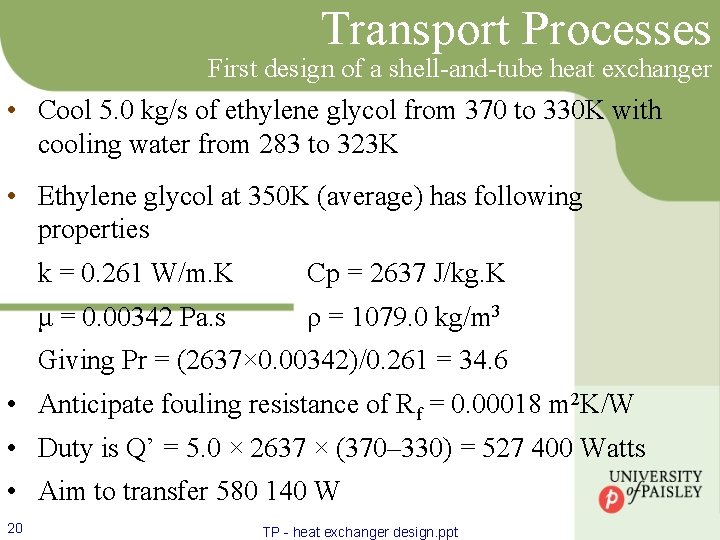
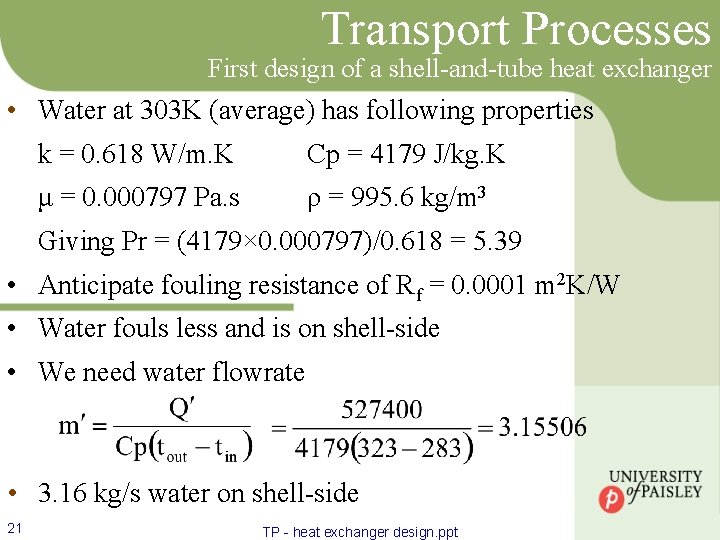
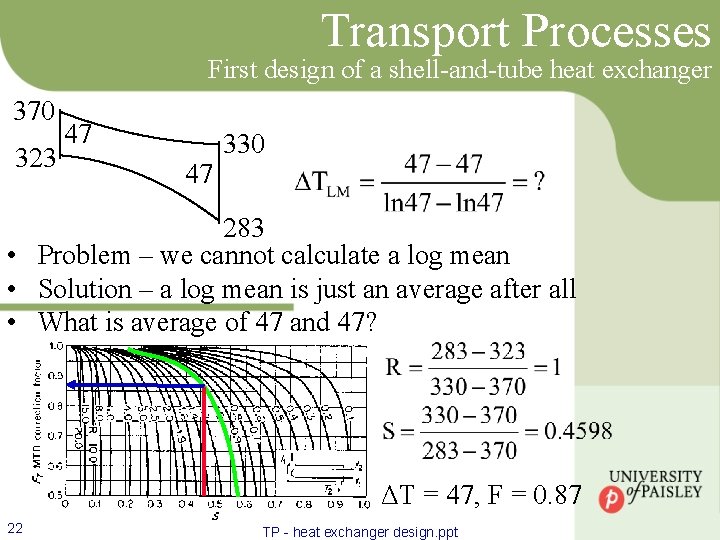
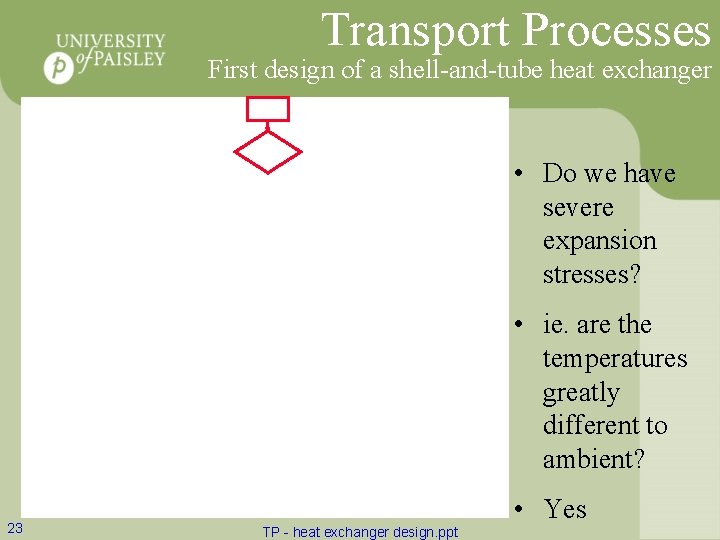
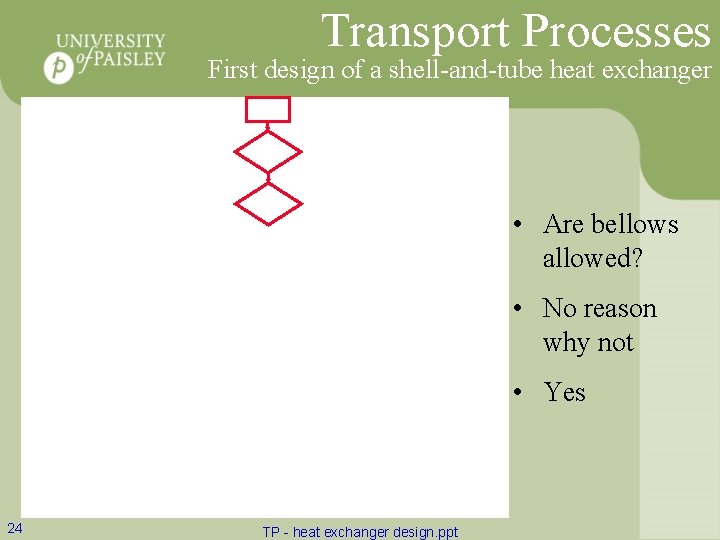
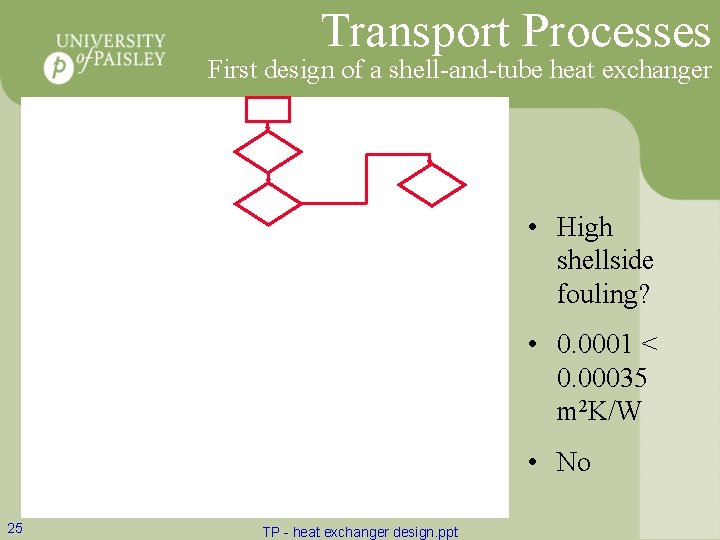
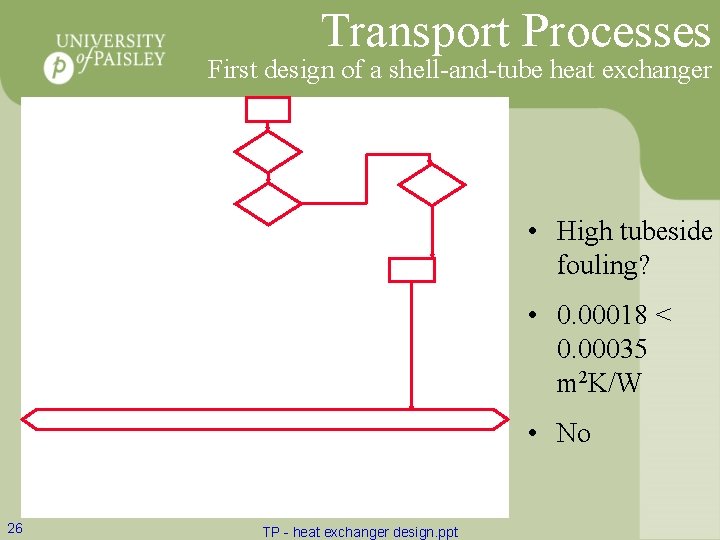
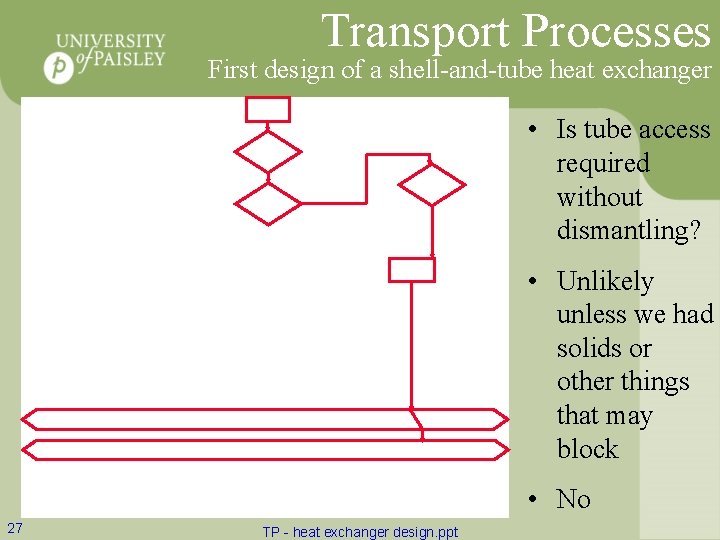
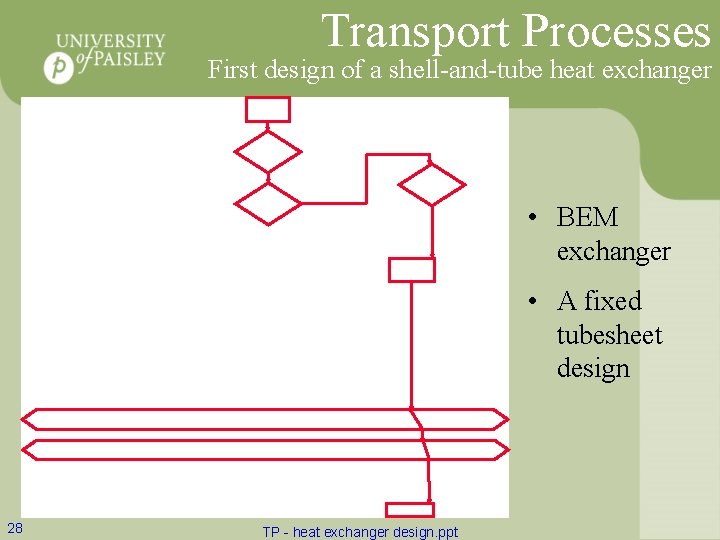
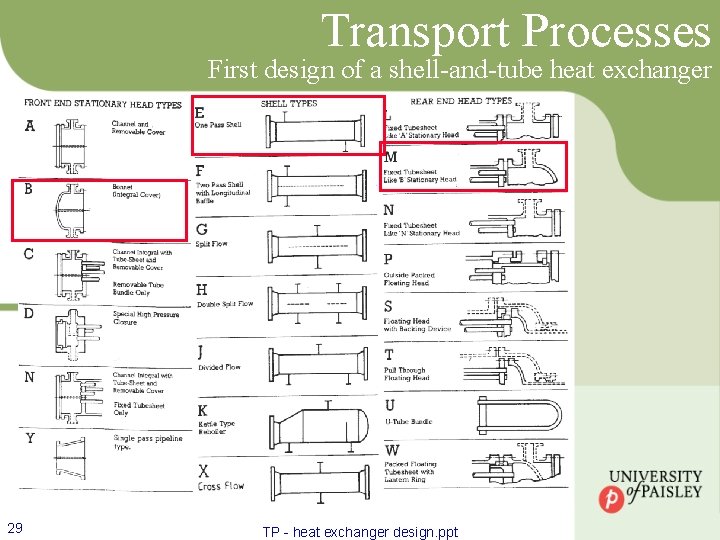
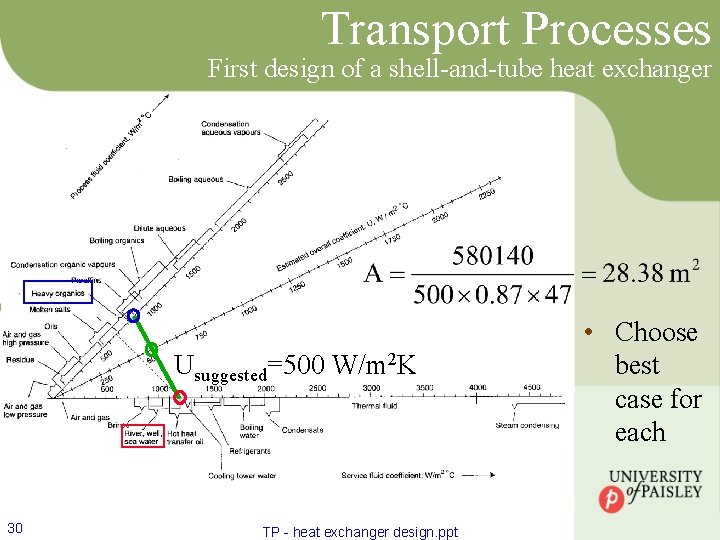
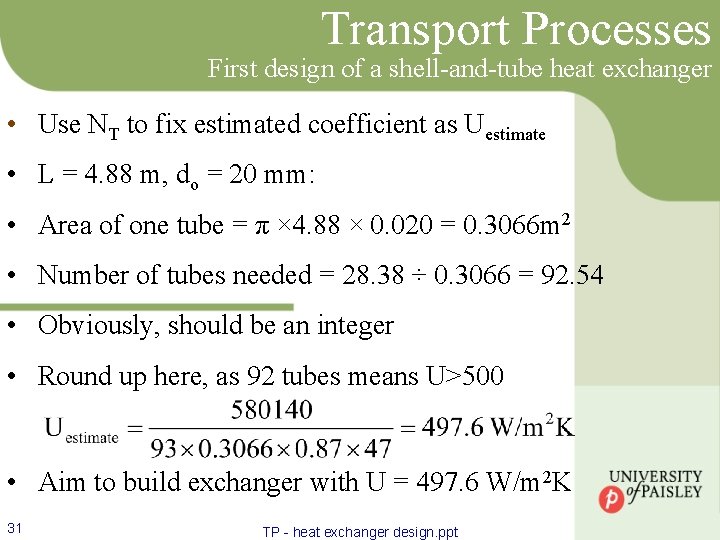
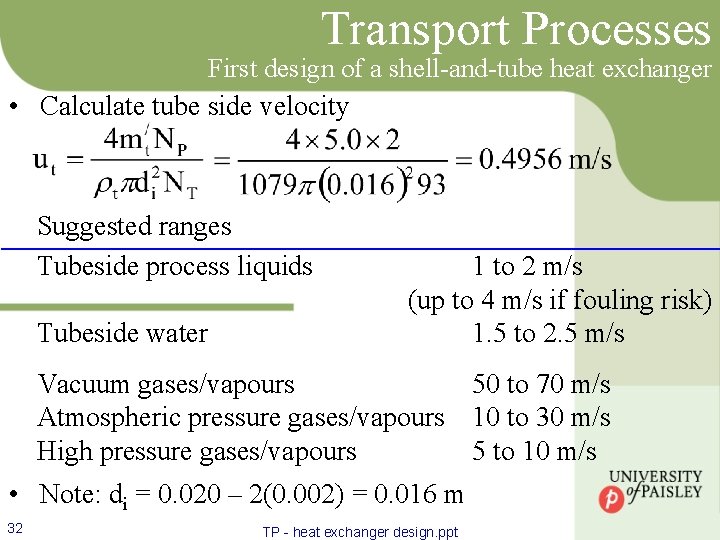
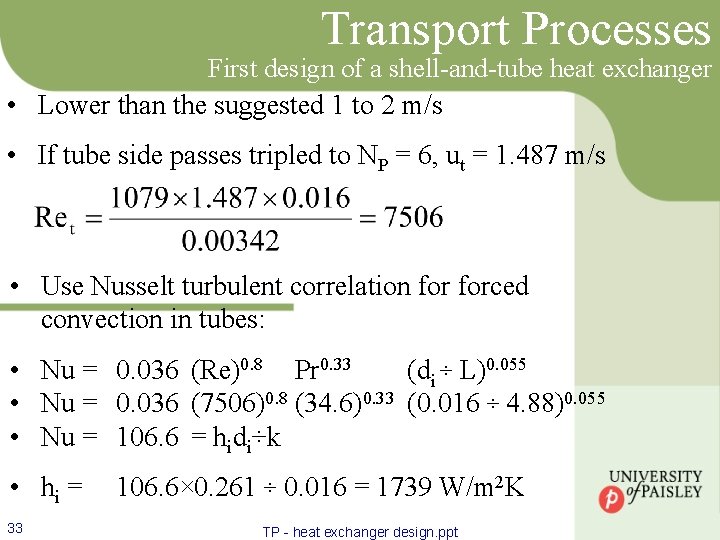
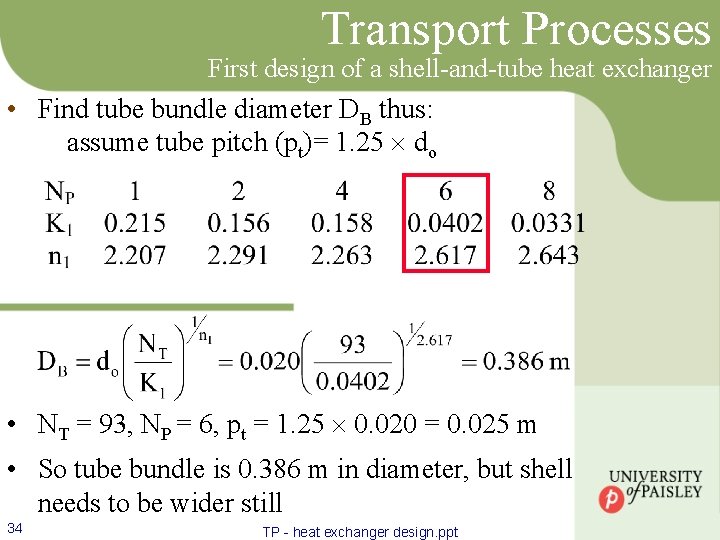
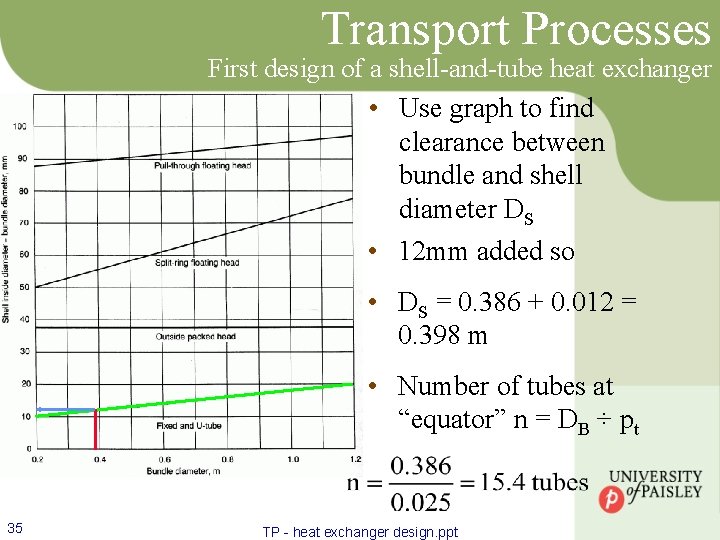
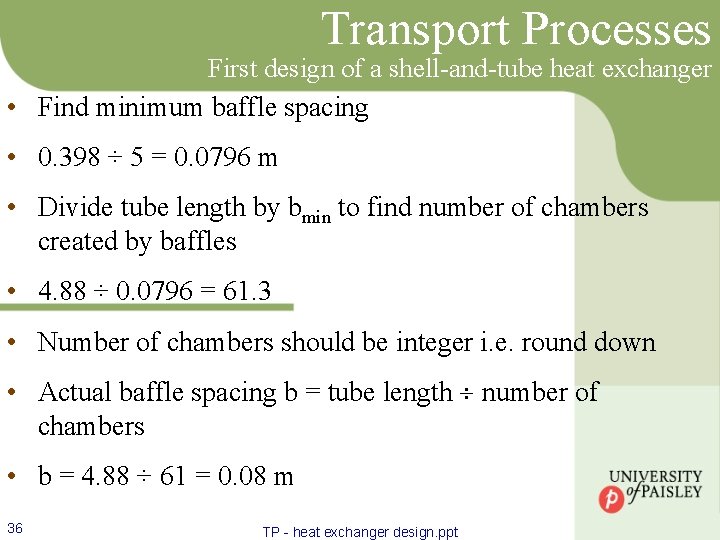
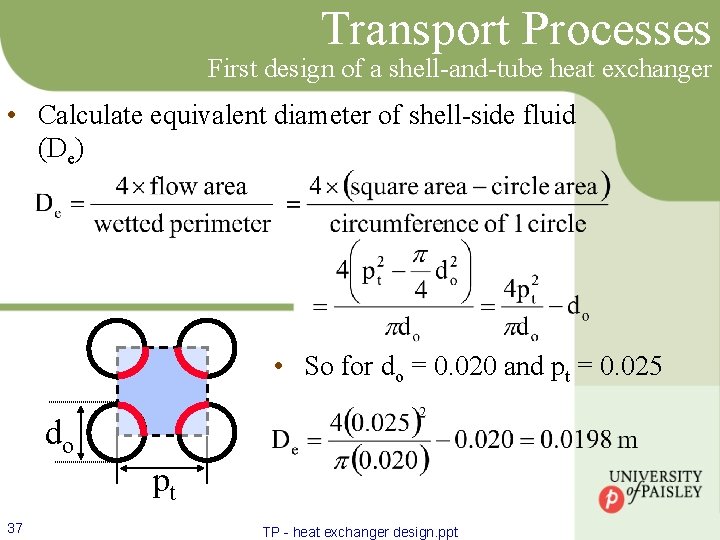
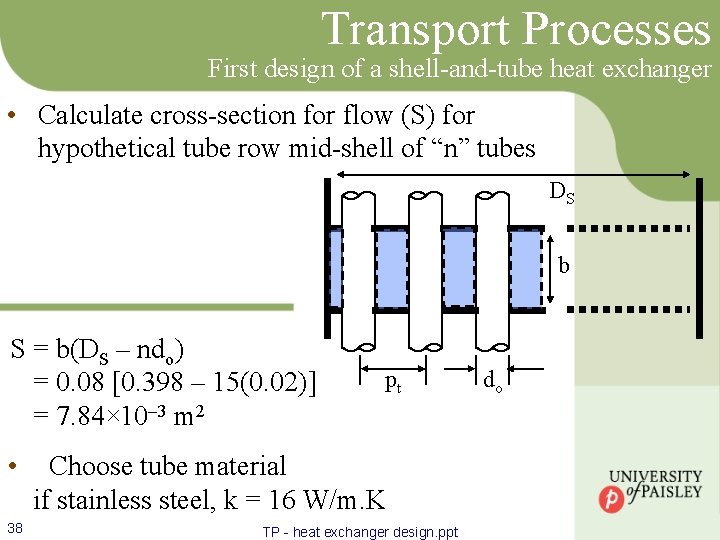
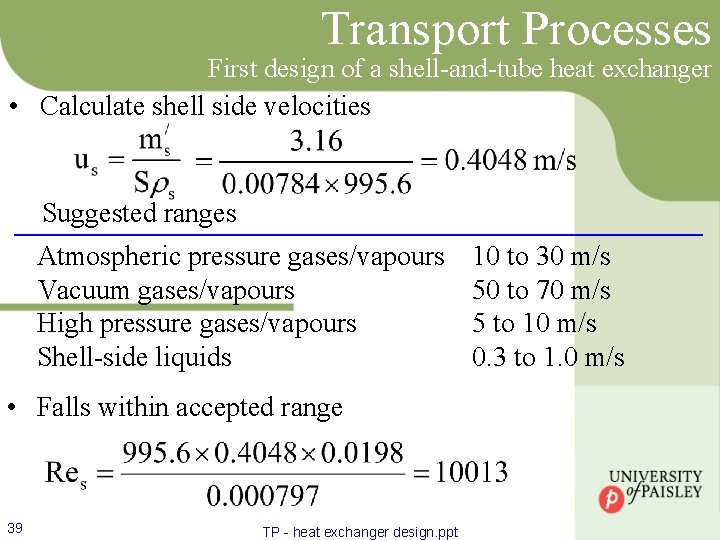
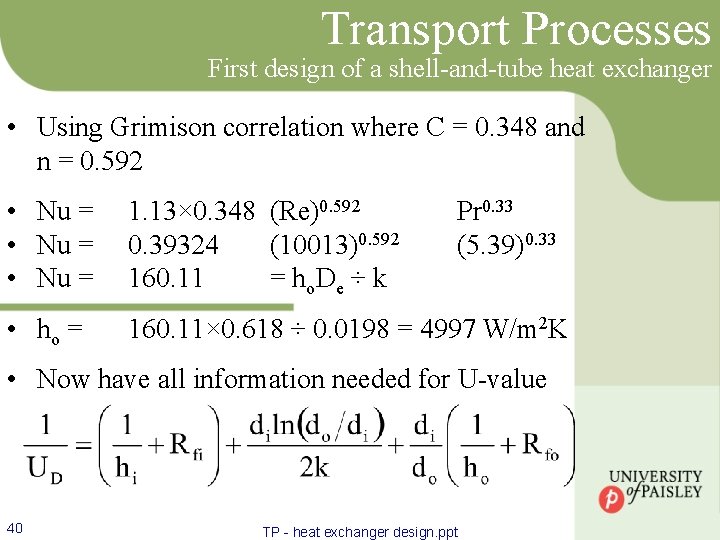
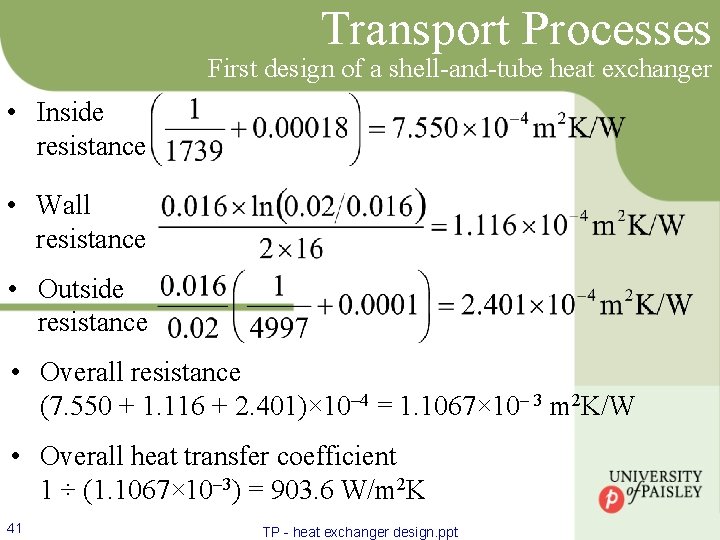
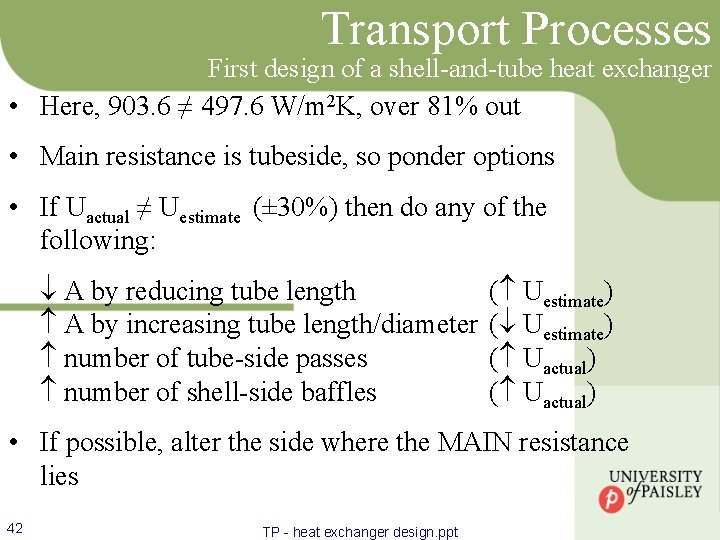
- Slides: 42
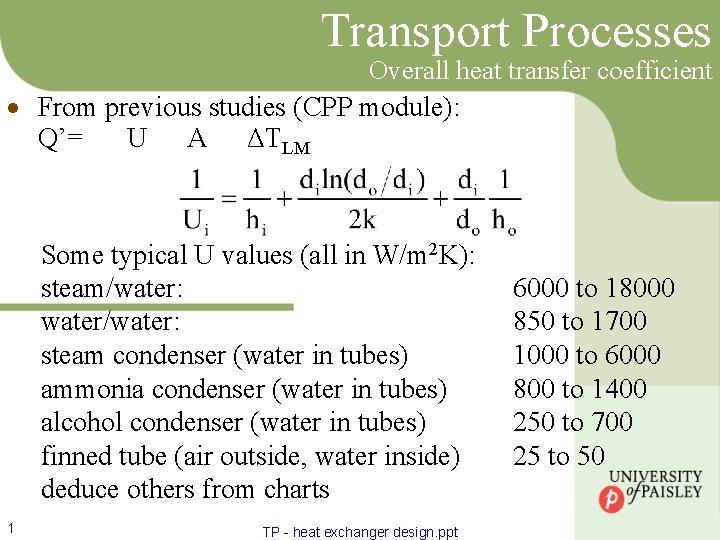
Transport Processes Overall heat transfer coefficient · From previous studies (CPP module): Q’= U A ΔTLM Some typical U values (all in W/m 2 K): steam/water: water/water: steam condenser (water in tubes) ammonia condenser (water in tubes) alcohol condenser (water in tubes) finned tube (air outside, water inside) deduce others from charts 1 TP - heat exchanger design. ppt 6000 to 18000 850 to 1700 1000 to 6000 800 to 1400 250 to 700 25 to 50
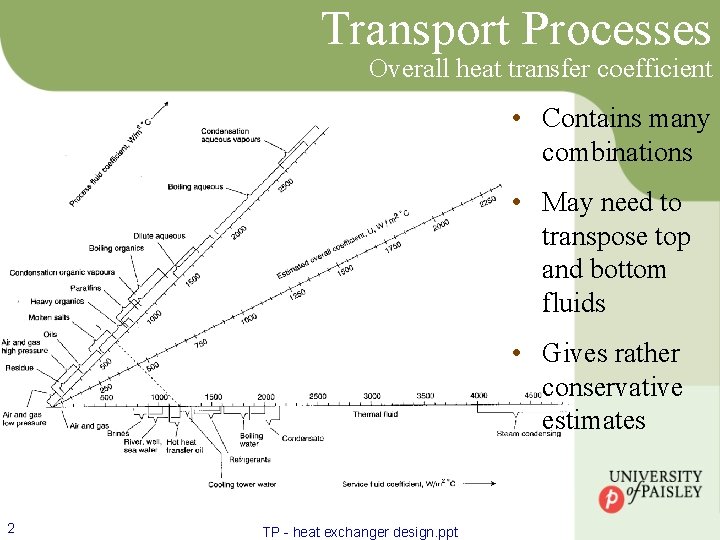
Transport Processes Overall heat transfer coefficient • Contains many combinations • May need to transpose top and bottom fluids • Gives rather conservative estimates 2 TP - heat exchanger design. ppt
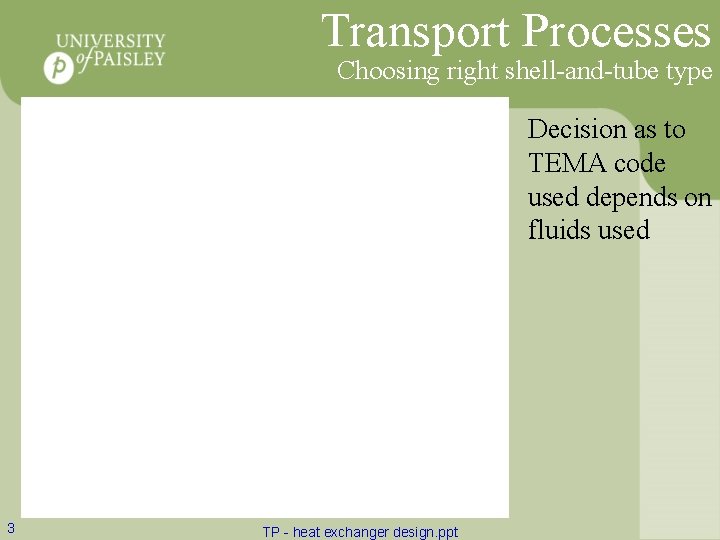
Transport Processes Choosing right shell-and-tube type Decision as to TEMA code used depends on fluids used 3 TP - heat exchanger design. ppt
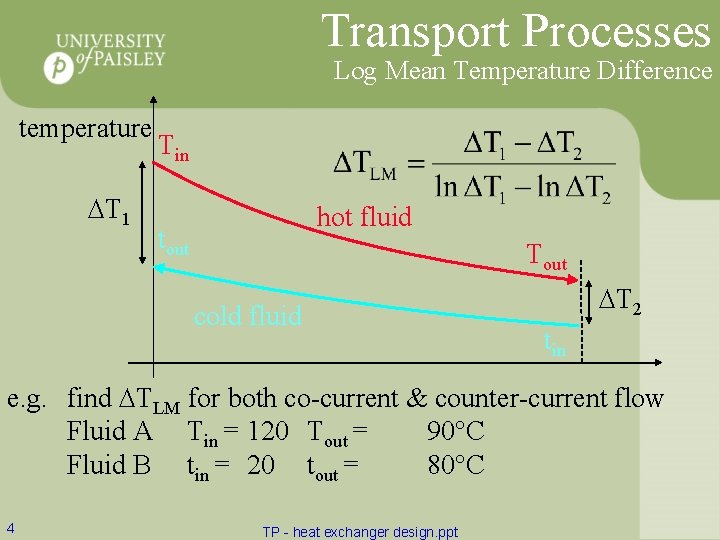
Transport Processes Log Mean Temperature Difference temperature T 1 Tin hot fluid tout Tout cold fluid T 2 tin e. g. find TLM for both co-current & counter-current flow Fluid A Tin = 120 Tout = 90°C Fluid B tin = 20 tout = 80°C 4 TP - heat exchanger design. ppt
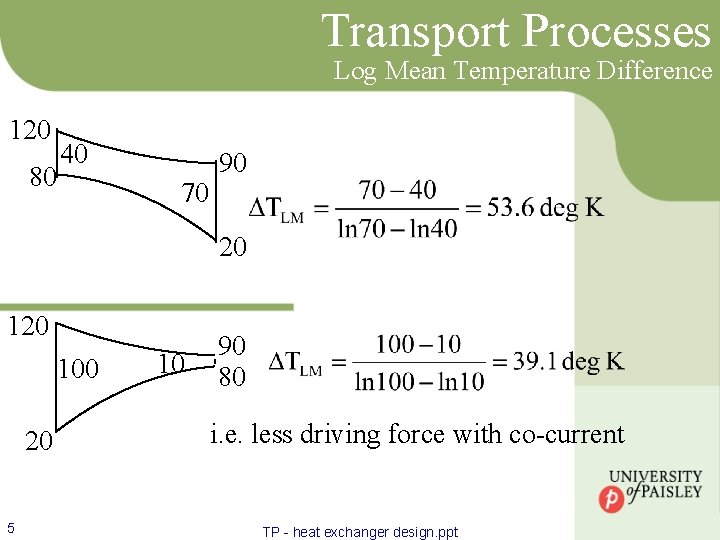
Transport Processes Log Mean Temperature Difference 120 80 40 70 90 20 100 20 5 10 90 80 i. e. less driving force with co-current TP - heat exchanger design. ppt
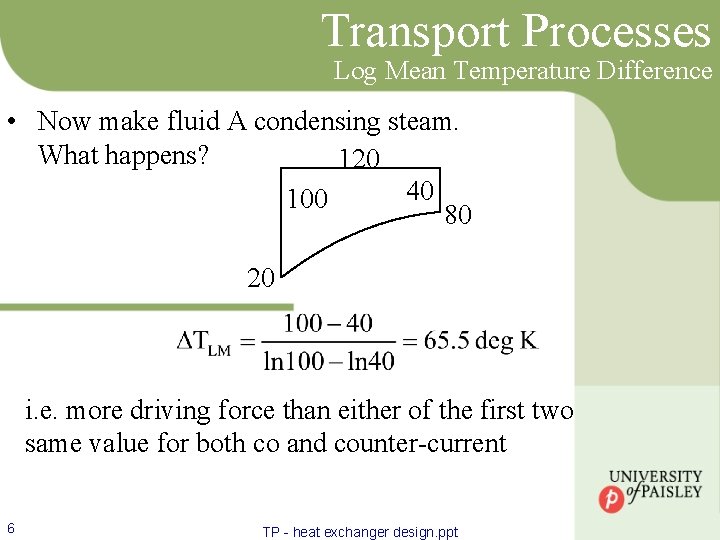
Transport Processes Log Mean Temperature Difference • Now make fluid A condensing steam. What happens? 120 40 100 80 20 i. e. more driving force than either of the first two same value for both co and counter-current 6 TP - heat exchanger design. ppt
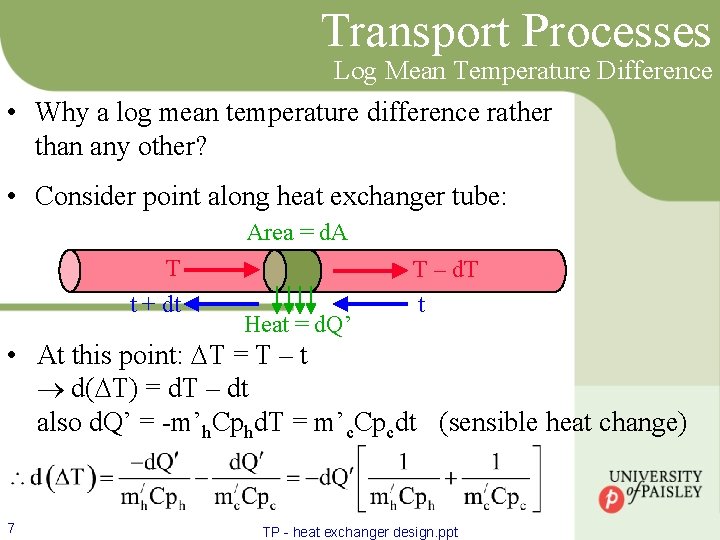
Transport Processes Log Mean Temperature Difference • Why a log mean temperature difference rather than any other? • Consider point along heat exchanger tube: Area = d. A T t + dt Heat = d. Q’ T – d. T t • At this point: T = T – t d( T) = d. T – dt also d. Q’ = -m’h. Cphd. T = m’c. Cpcdt (sensible heat change) 7 TP - heat exchanger design. ppt
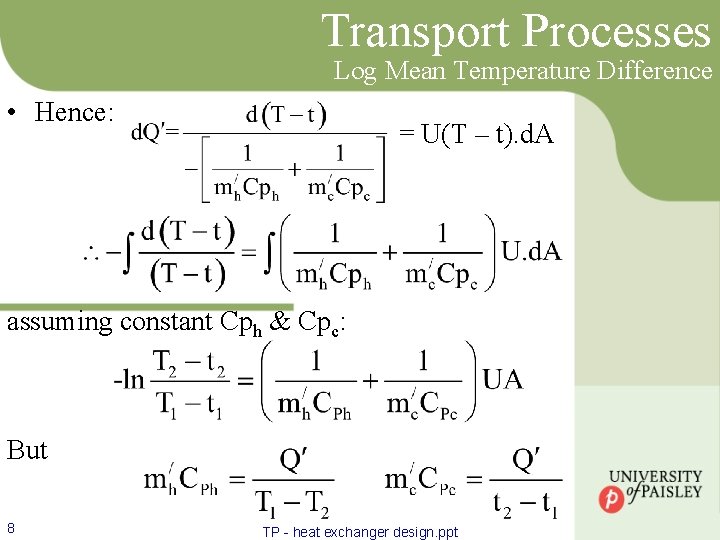
Transport Processes Log Mean Temperature Difference • Hence: = U(T – t). d. A assuming constant Cph & Cpc: But 8 TP - heat exchanger design. ppt
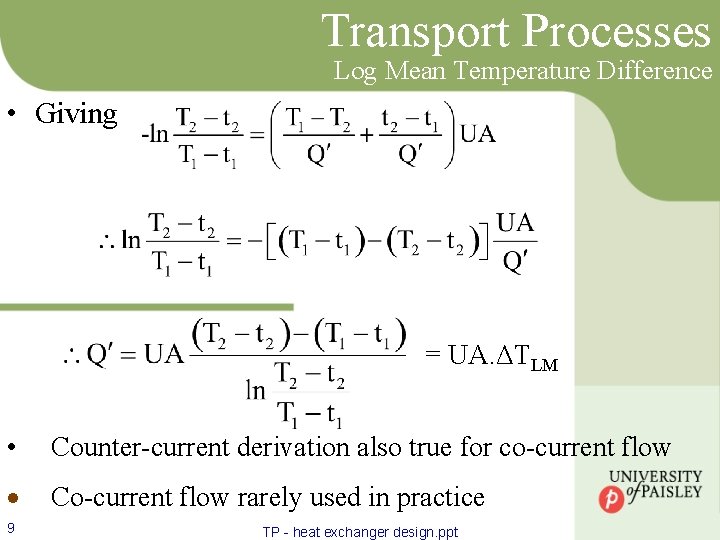
Transport Processes Log Mean Temperature Difference • Giving = UA. ΔTLM • Counter-current derivation also true for co-current flow · Co-current flow rarely used in practice 9 TP - heat exchanger design. ppt
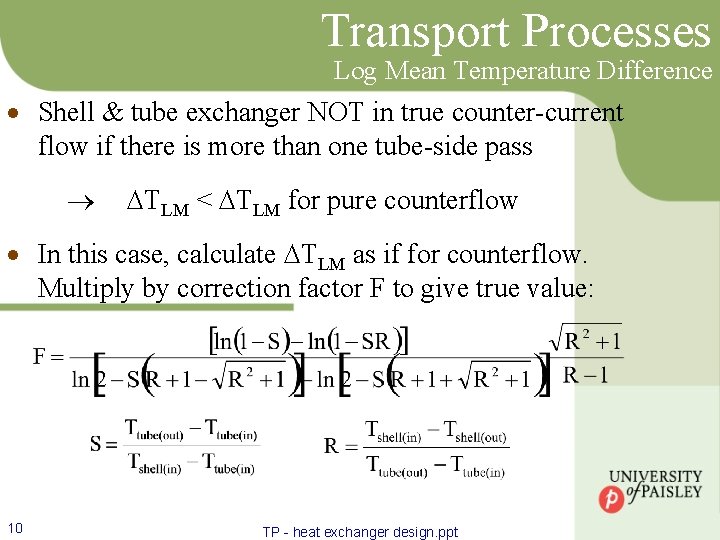
Transport Processes Log Mean Temperature Difference · Shell & tube exchanger NOT in true counter-current flow if there is more than one tube-side pass TLM < TLM for pure counterflow · In this case, calculate TLM as if for counterflow. Multiply by correction factor F to give true value: 10 TP - heat exchanger design. ppt
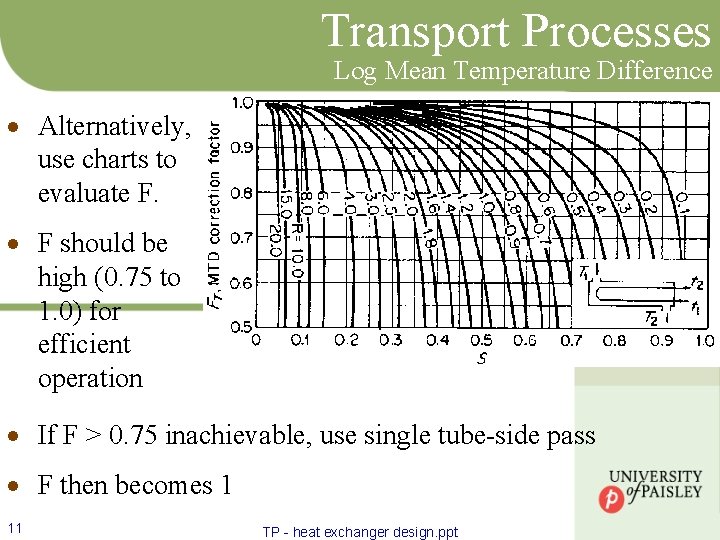
Transport Processes Log Mean Temperature Difference · Alternatively, use charts to evaluate F. · F should be high (0. 75 to 1. 0) for efficient operation · If F > 0. 75 inachievable, use single tube-side pass · F then becomes 1 11 TP - heat exchanger design. ppt
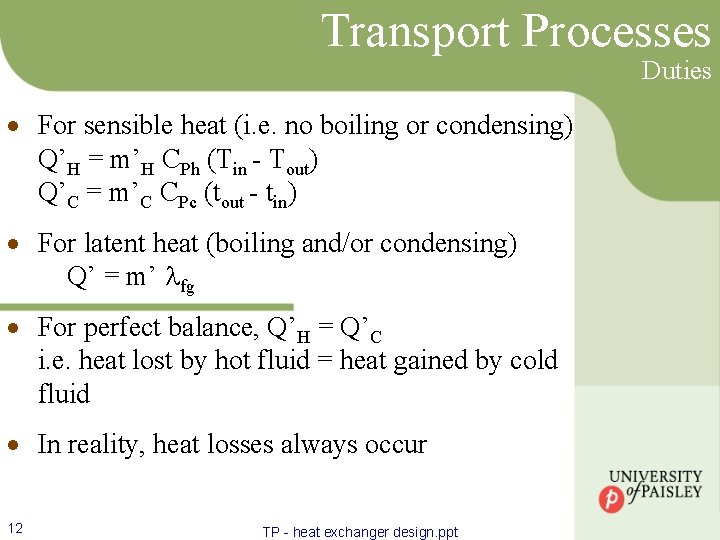
Transport Processes Duties · For sensible heat (i. e. no boiling or condensing) Q’H = m’H CPh (Tin - Tout) Q’C = m’C CPc (tout - tin) · For latent heat (boiling and/or condensing) Q’ = m’ fg · For perfect balance, Q’H = Q’C i. e. heat lost by hot fluid = heat gained by cold fluid · In reality, heat losses always occur 12 TP - heat exchanger design. ppt
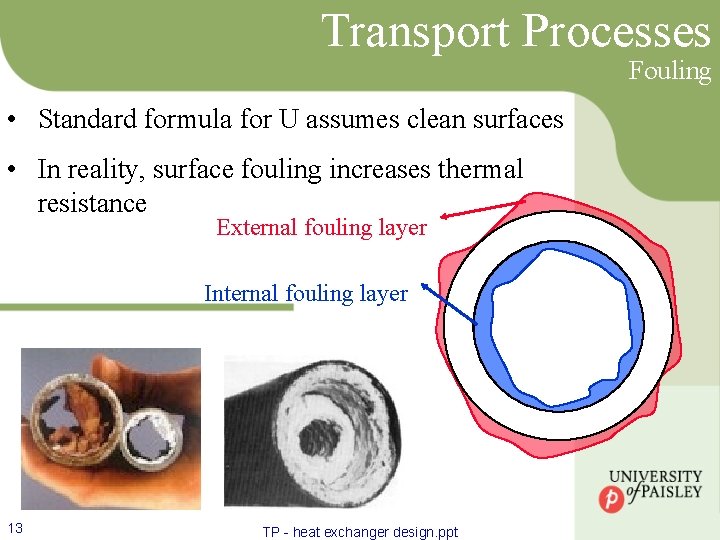
Transport Processes Fouling • Standard formula for U assumes clean surfaces • In reality, surface fouling increases thermal resistance External fouling layer Internal fouling layer 13 TP - heat exchanger design. ppt
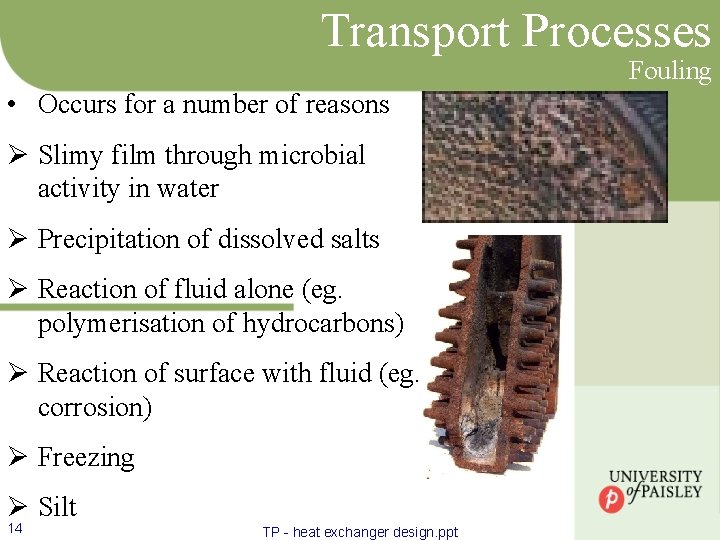
Transport Processes Fouling • Occurs for a number of reasons Ø Slimy film through microbial activity in water Ø Precipitation of dissolved salts Ø Reaction of fluid alone (eg. polymerisation of hydrocarbons) Ø Reaction of surface with fluid (eg. corrosion) Ø Freezing Ø Silt 14 TP - heat exchanger design. ppt
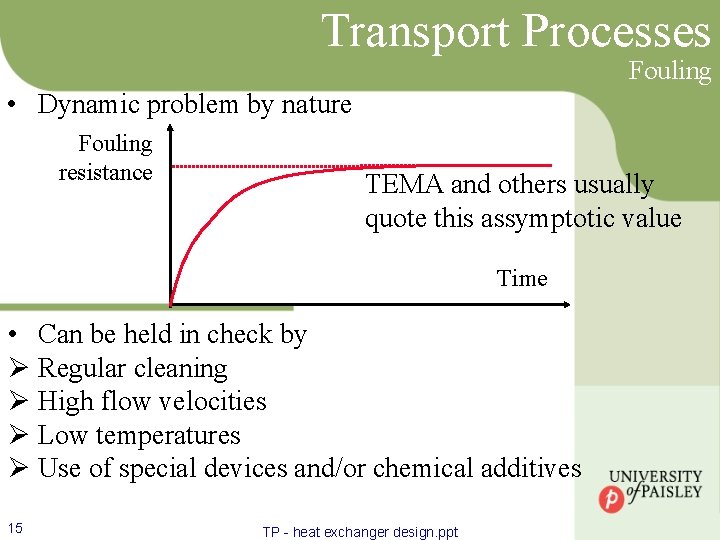
Transport Processes Fouling • Dynamic problem by nature Fouling resistance TEMA and others usually quote this assymptotic value Time • Can be held in check by Ø Regular cleaning Ø High flow velocities Ø Low temperatures Ø Use of special devices and/or chemical additives 15 TP - heat exchanger design. ppt
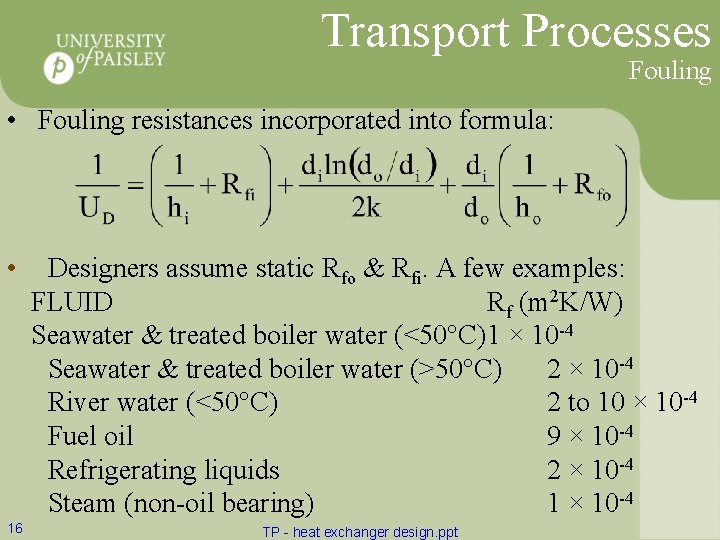
Transport Processes Fouling • Fouling resistances incorporated into formula: • 16 Designers assume static Rfo & Rfi. A few examples: FLUID Rf (m 2 K/W) Seawater & treated boiler water (<50°C)1 × 10 -4 Seawater & treated boiler water (>50°C) 2 × 10 -4 River water (<50°C) 2 to 10 × 10 -4 Fuel oil 9 × 10 -4 Refrigerating liquids 2 × 10 -4 Steam (non-oil bearing) 1 × 10 -4 TP - heat exchanger design. ppt
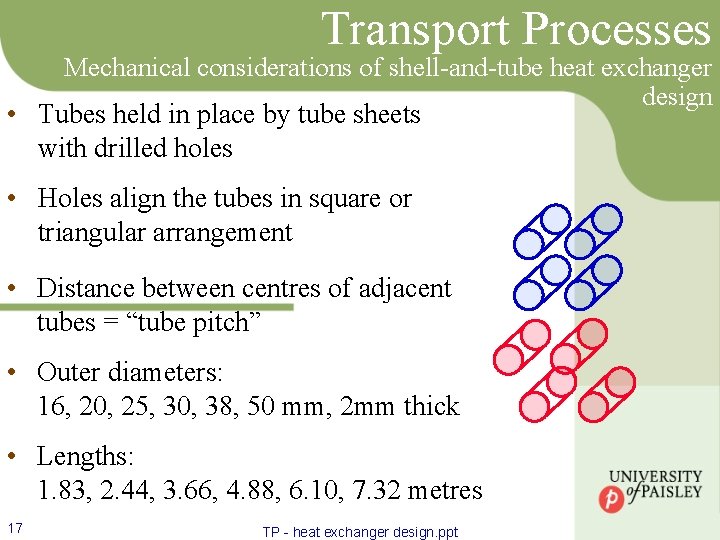
Transport Processes Mechanical considerations of shell-and-tube heat exchanger design • Tubes held in place by tube sheets with drilled holes • Holes align the tubes in square or triangular arrangement • Distance between centres of adjacent tubes = “tube pitch” • Outer diameters: 16, 20, 25, 30, 38, 50 mm, 2 mm thick • Lengths: 1. 83, 2. 44, 3. 66, 4. 88, 6. 10, 7. 32 metres 17 TP - heat exchanger design. ppt
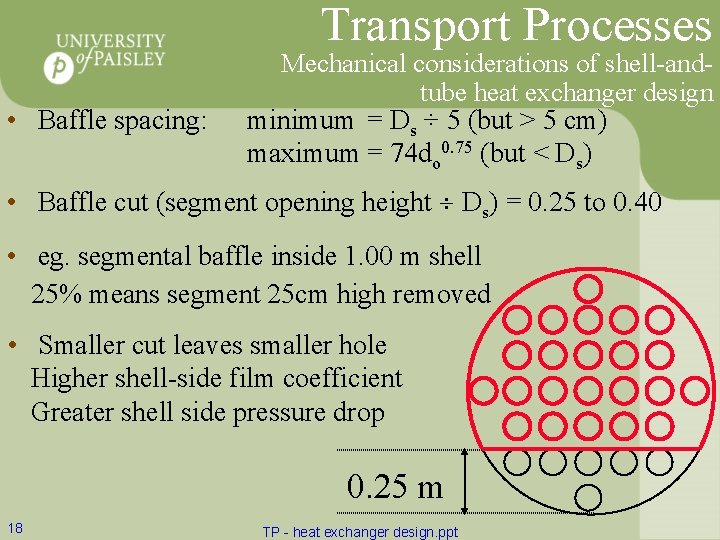
Transport Processes • Baffle spacing: Mechanical considerations of shell-andtube heat exchanger design minimum = Ds ÷ 5 (but > 5 cm) maximum = 74 do 0. 75 (but < Ds) • Baffle cut (segment opening height Ds) = 0. 25 to 0. 40 • eg. segmental baffle inside 1. 00 m shell 25% means segment 25 cm high removed • Smaller cut leaves smaller hole Higher shell-side film coefficient Greater shell side pressure drop 0. 25 m 18 TP - heat exchanger design. ppt
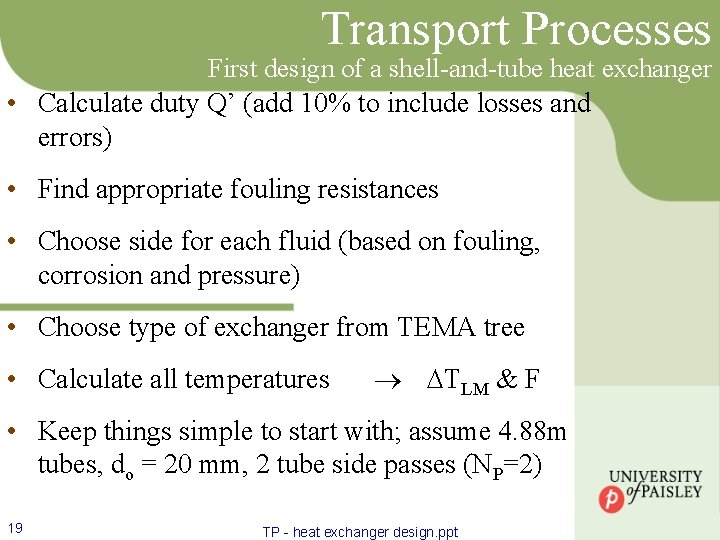
Transport Processes First design of a shell-and-tube heat exchanger • Calculate duty Q’ (add 10% to include losses and errors) • Find appropriate fouling resistances • Choose side for each fluid (based on fouling, corrosion and pressure) • Choose type of exchanger from TEMA tree • Calculate all temperatures TLM & F • Keep things simple to start with; assume 4. 88 m tubes, do = 20 mm, 2 tube side passes (NP=2) 19 TP - heat exchanger design. ppt
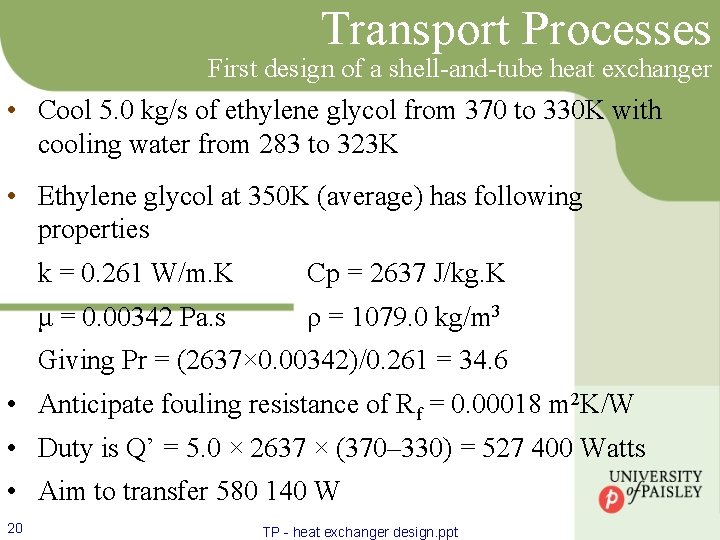
Transport Processes First design of a shell-and-tube heat exchanger • Cool 5. 0 kg/s of ethylene glycol from 370 to 330 K with cooling water from 283 to 323 K • Ethylene glycol at 350 K (average) has following properties k = 0. 261 W/m. K Cp = 2637 J/kg. K μ = 0. 00342 Pa. s ρ = 1079. 0 kg/m 3 Giving Pr = (2637× 0. 00342)/0. 261 = 34. 6 • Anticipate fouling resistance of Rf = 0. 00018 m 2 K/W • Duty is Q’ = 5. 0 × 2637 × (370– 330) = 527 400 Watts • Aim to transfer 580 140 W 20 TP - heat exchanger design. ppt
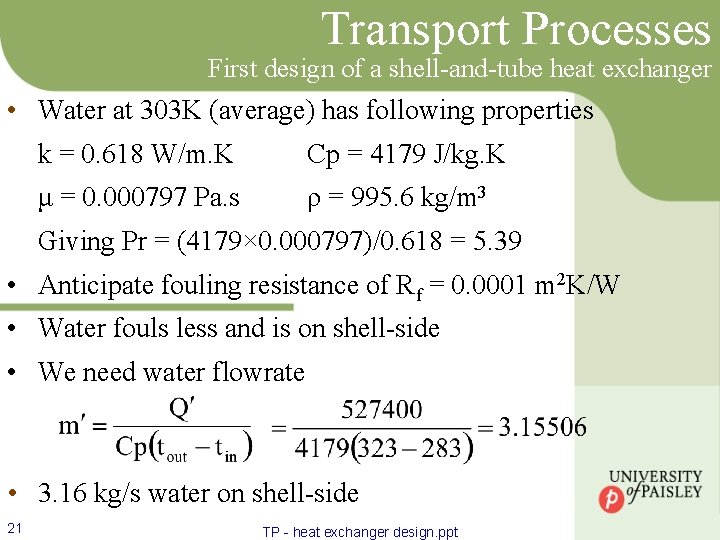
Transport Processes First design of a shell-and-tube heat exchanger • Water at 303 K (average) has following properties k = 0. 618 W/m. K Cp = 4179 J/kg. K μ = 0. 000797 Pa. s ρ = 995. 6 kg/m 3 Giving Pr = (4179× 0. 000797)/0. 618 = 5. 39 • Anticipate fouling resistance of Rf = 0. 0001 m 2 K/W • Water fouls less and is on shell-side • We need water flowrate • 3. 16 kg/s water on shell-side 21 TP - heat exchanger design. ppt
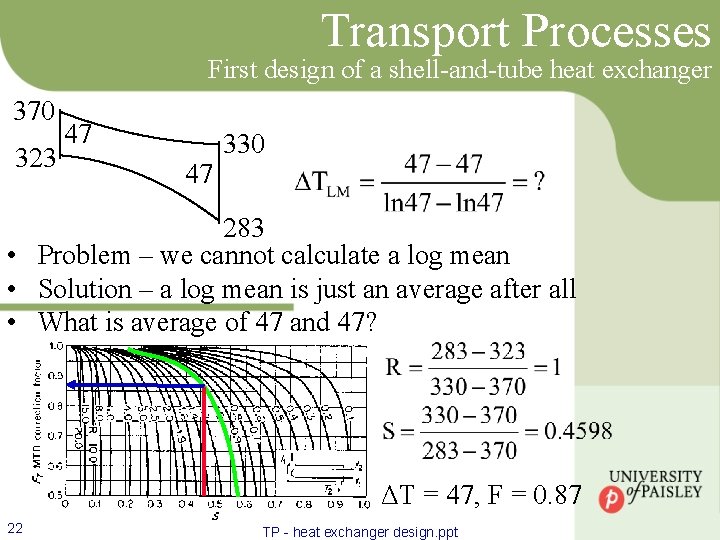
Transport Processes First design of a shell-and-tube heat exchanger 370 323 47 47 330 283 • Problem – we cannot calculate a log mean • Solution – a log mean is just an average after all • What is average of 47 and 47? ΔT = 47, F = 0. 87 22 TP - heat exchanger design. ppt
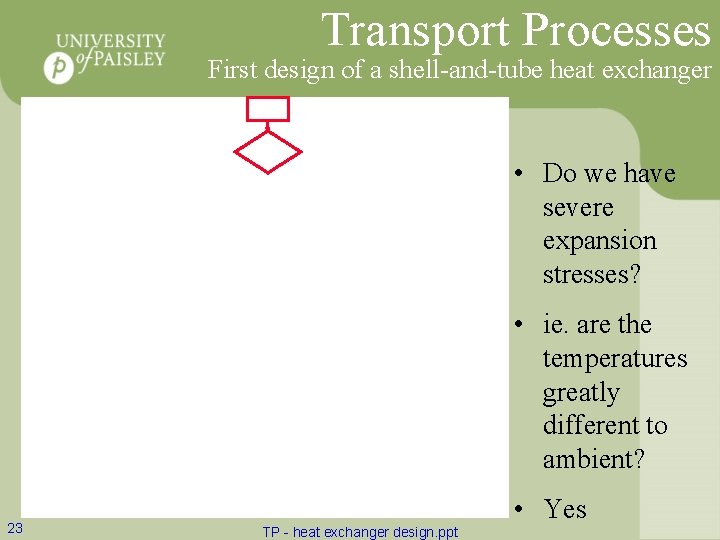
Transport Processes First design of a shell-and-tube heat exchanger • Do we have severe expansion stresses? • ie. are the temperatures greatly different to ambient? 23 • Yes TP - heat exchanger design. ppt
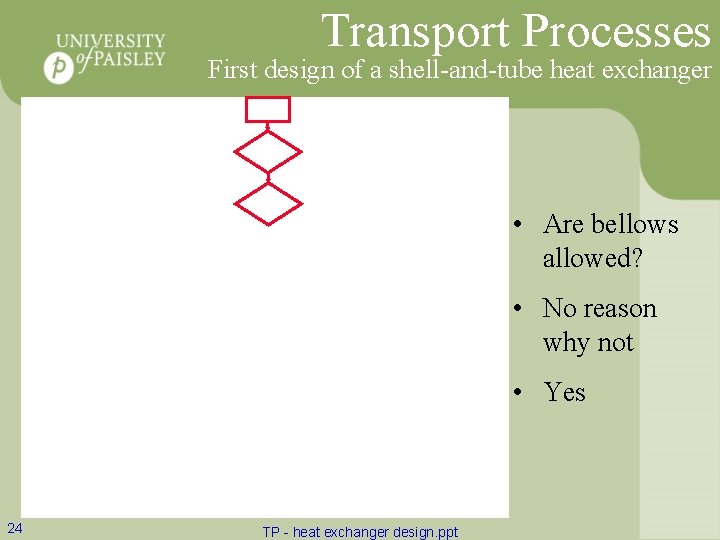
Transport Processes First design of a shell-and-tube heat exchanger • Are bellows allowed? • No reason why not • Yes 24 TP - heat exchanger design. ppt
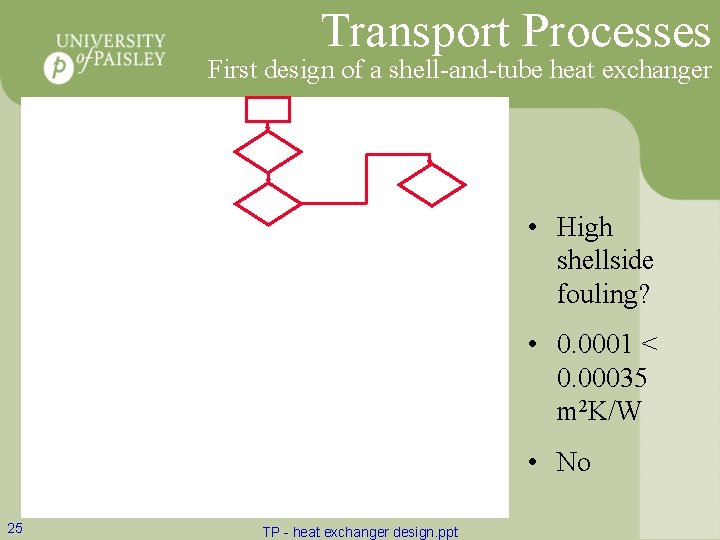
Transport Processes First design of a shell-and-tube heat exchanger • High shellside fouling? • 0. 0001 < 0. 00035 m 2 K/W • No 25 TP - heat exchanger design. ppt
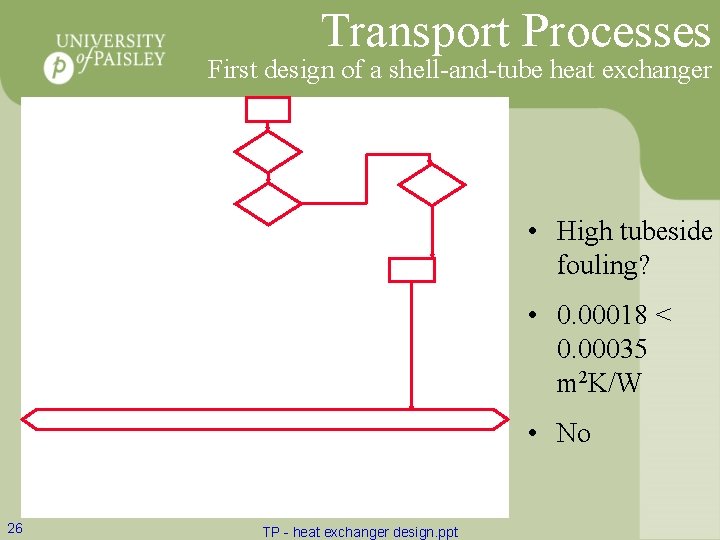
Transport Processes First design of a shell-and-tube heat exchanger • High tubeside fouling? • 0. 00018 < 0. 00035 m 2 K/W • No 26 TP - heat exchanger design. ppt
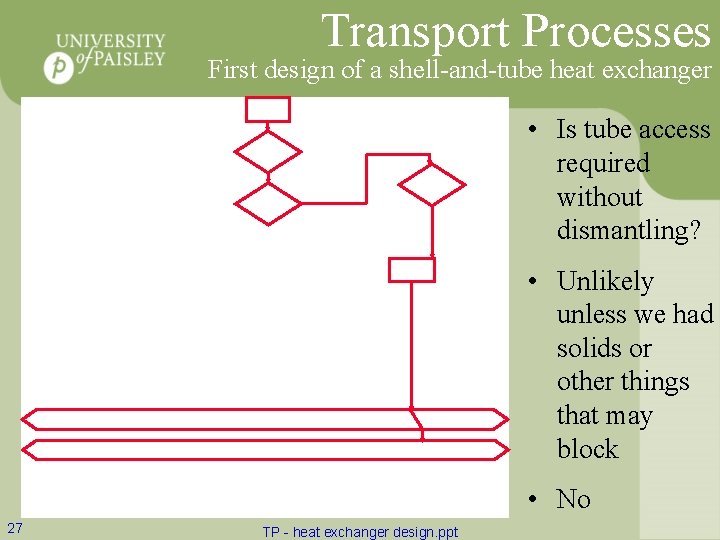
Transport Processes First design of a shell-and-tube heat exchanger • Is tube access required without dismantling? • Unlikely unless we had solids or other things that may block • No 27 TP - heat exchanger design. ppt
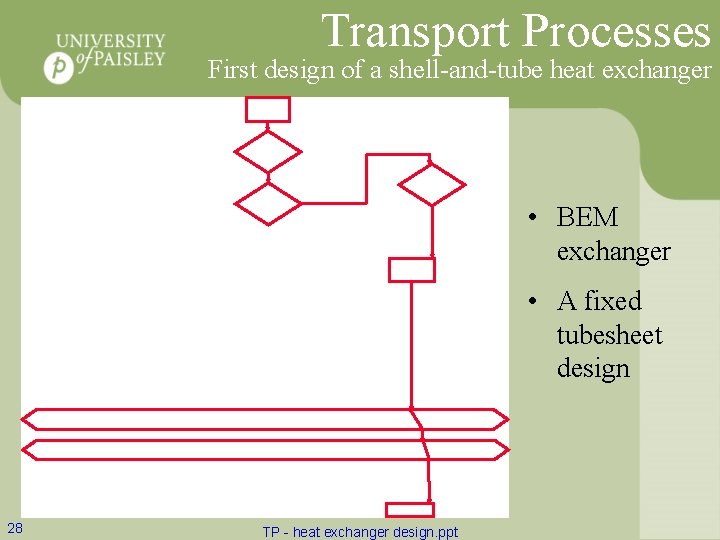
Transport Processes First design of a shell-and-tube heat exchanger • BEM exchanger • A fixed tubesheet design 28 TP - heat exchanger design. ppt
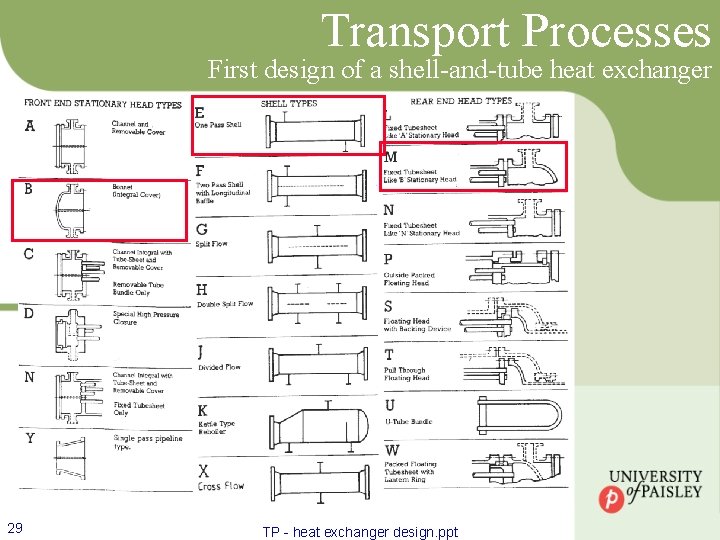
Transport Processes First design of a shell-and-tube heat exchanger 29 TP - heat exchanger design. ppt
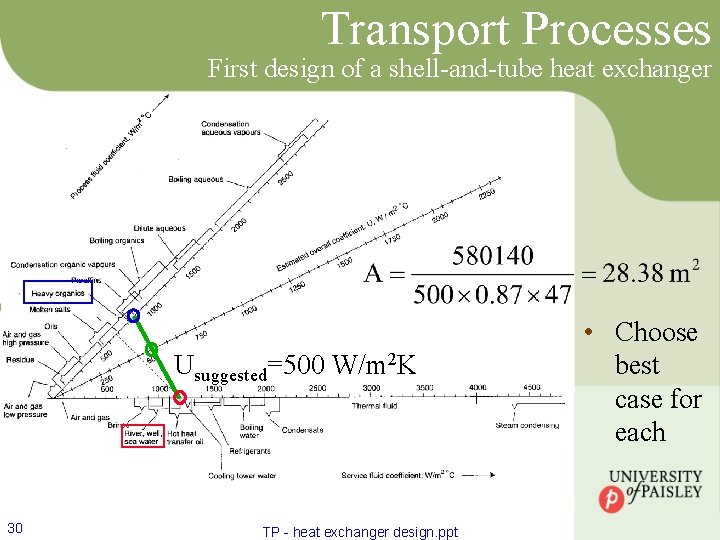
Transport Processes First design of a shell-and-tube heat exchanger Usuggested=500 W/m 2 K 30 TP - heat exchanger design. ppt • Choose best case for each
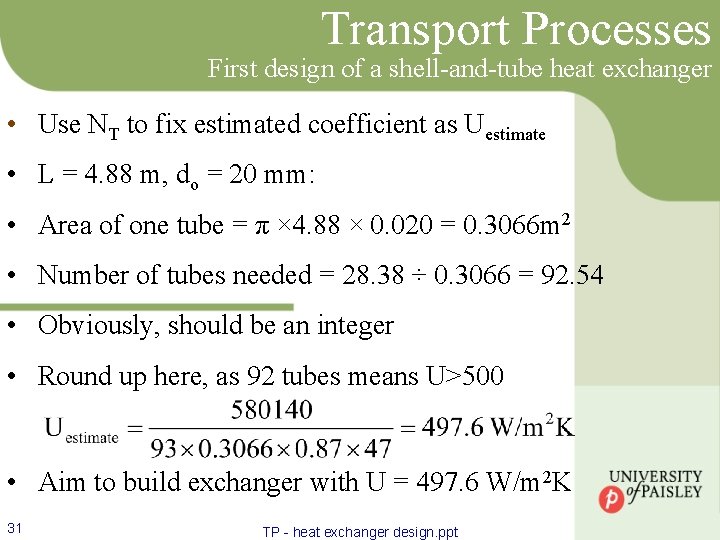
Transport Processes First design of a shell-and-tube heat exchanger • Use NT to fix estimated coefficient as Uestimate • L = 4. 88 m, do = 20 mm: • Area of one tube = π × 4. 88 × 0. 020 = 0. 3066 m 2 • Number of tubes needed = 28. 38 ÷ 0. 3066 = 92. 54 • Obviously, should be an integer • Round up here, as 92 tubes means U>500 • Aim to build exchanger with U = 497. 6 W/m 2 K 31 TP - heat exchanger design. ppt
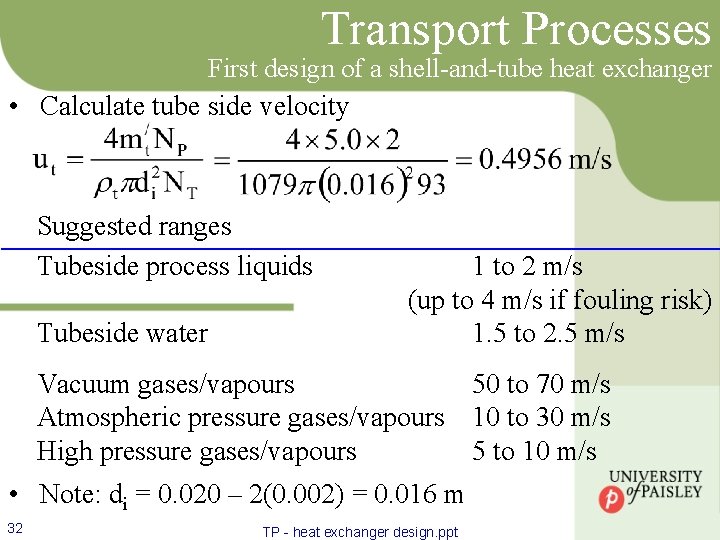
Transport Processes First design of a shell-and-tube heat exchanger • Calculate tube side velocity Suggested ranges Tubeside process liquids Tubeside water 1 to 2 m/s (up to 4 m/s if fouling risk) 1. 5 to 2. 5 m/s Vacuum gases/vapours 50 to 70 m/s Atmospheric pressure gases/vapours 10 to 30 m/s High pressure gases/vapours 5 to 10 m/s • Note: di = 0. 020 – 2(0. 002) = 0. 016 m 32 TP - heat exchanger design. ppt
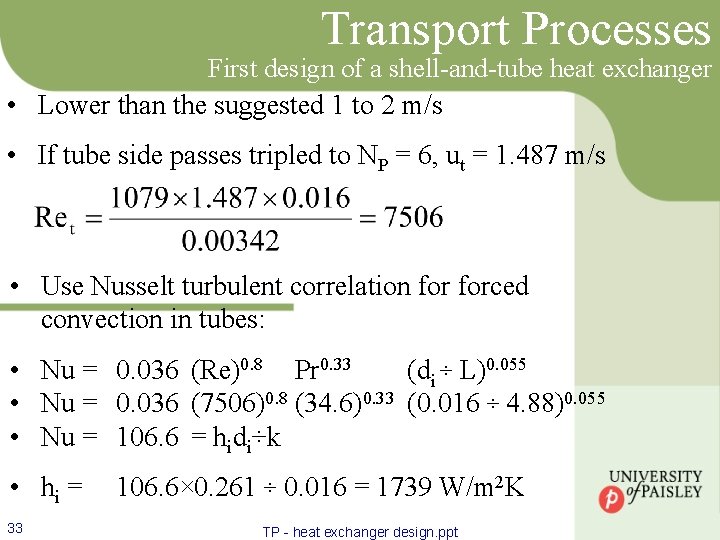
Transport Processes First design of a shell-and-tube heat exchanger • Lower than the suggested 1 to 2 m/s • If tube side passes tripled to NP = 6, ut = 1. 487 m/s • Use Nusselt turbulent correlation forced convection in tubes: • Nu = 0. 036 (Re)0. 8 Pr 0. 33 (di ÷ L)0. 055 • Nu = 0. 036 (7506)0. 8 (34. 6)0. 33 (0. 016 ÷ 4. 88)0. 055 • Nu = 106. 6 = hidi÷k • hi = 33 106. 6× 0. 261 ÷ 0. 016 = 1739 W/m 2 K TP - heat exchanger design. ppt
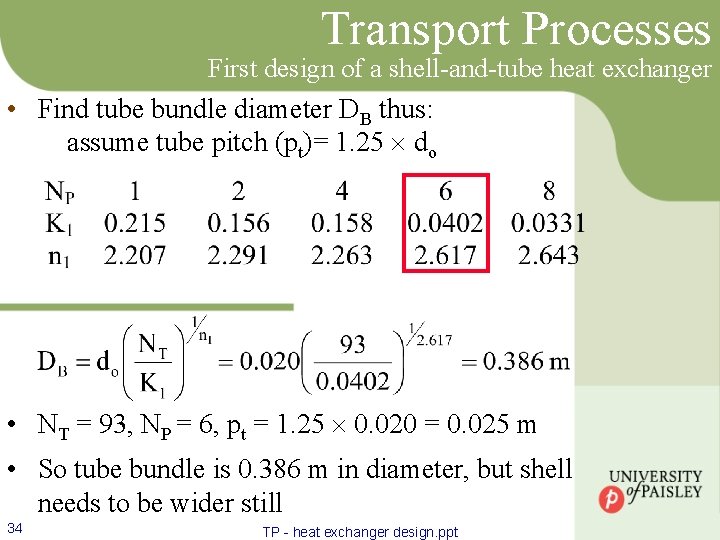
Transport Processes First design of a shell-and-tube heat exchanger • Find tube bundle diameter DB thus: assume tube pitch (pt)= 1. 25 do • NT = 93, NP = 6, pt = 1. 25 0. 020 = 0. 025 m • So tube bundle is 0. 386 m in diameter, but shell needs to be wider still 34 TP - heat exchanger design. ppt
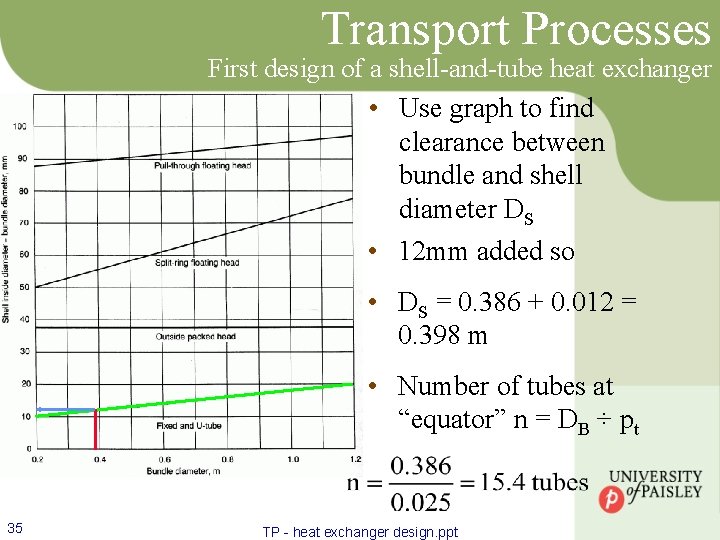
Transport Processes First design of a shell-and-tube heat exchanger • Use graph to find clearance between bundle and shell diameter DS • 12 mm added so • DS = 0. 386 + 0. 012 = 0. 398 m • Number of tubes at “equator” n = DB ÷ pt 35 TP - heat exchanger design. ppt
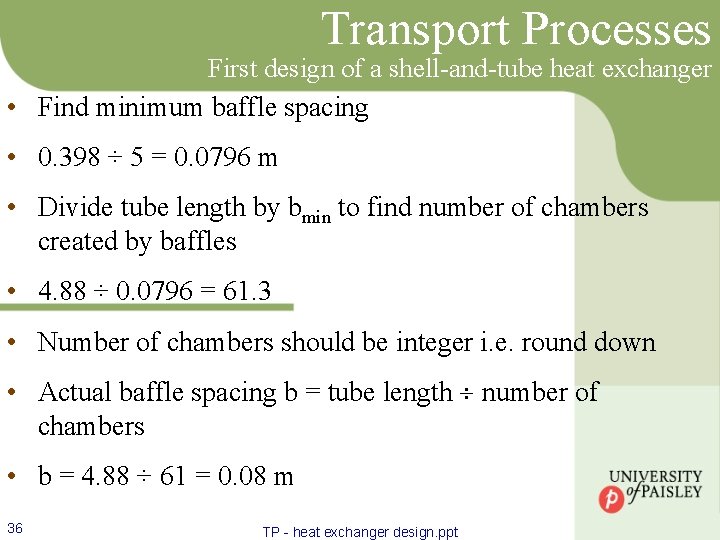
Transport Processes First design of a shell-and-tube heat exchanger • Find minimum baffle spacing • 0. 398 ÷ 5 = 0. 0796 m • Divide tube length by bmin to find number of chambers created by baffles • 4. 88 ÷ 0. 0796 = 61. 3 • Number of chambers should be integer i. e. round down • Actual baffle spacing b = tube length number of chambers • b = 4. 88 ÷ 61 = 0. 08 m 36 TP - heat exchanger design. ppt
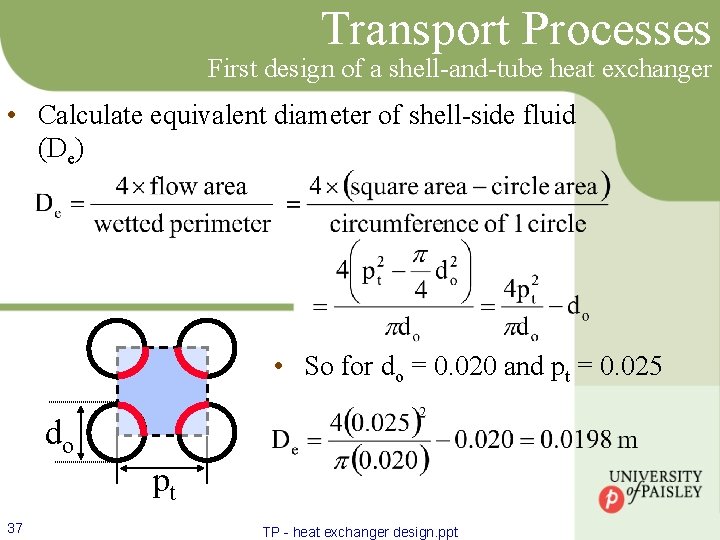
Transport Processes First design of a shell-and-tube heat exchanger • Calculate equivalent diameter of shell-side fluid (De) • So for do = 0. 020 and pt = 0. 025 do pt 37 TP - heat exchanger design. ppt
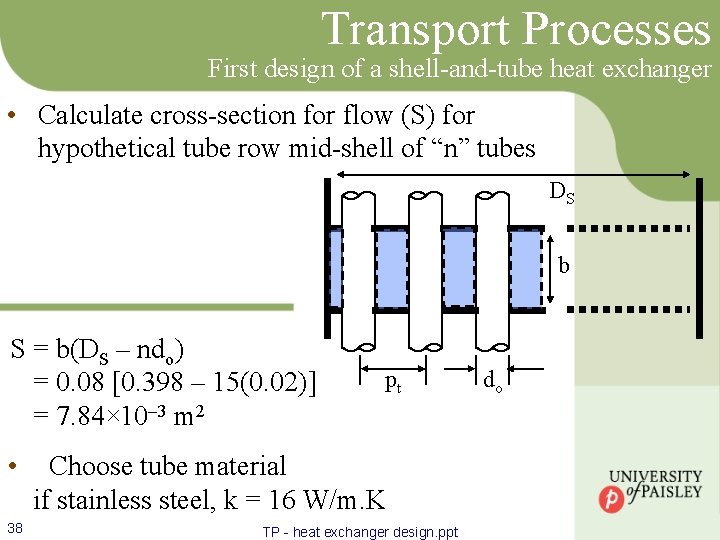
Transport Processes First design of a shell-and-tube heat exchanger • Calculate cross-section for flow (S) for hypothetical tube row mid-shell of “n” tubes DS b S = b(DS – ndo) = 0. 08 [0. 398 – 15(0. 02)] = 7. 84× 10– 3 m 2 • 38 pt Choose tube material if stainless steel, k = 16 W/m. K TP - heat exchanger design. ppt do
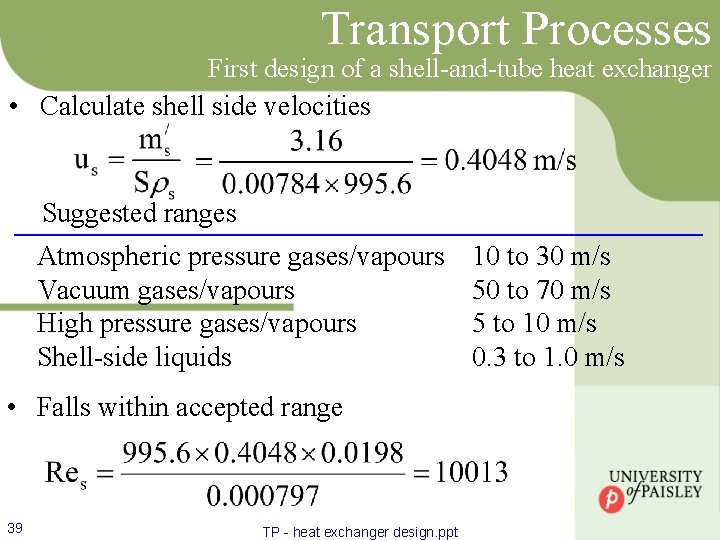
Transport Processes First design of a shell-and-tube heat exchanger • Calculate shell side velocities Suggested ranges Atmospheric pressure gases/vapours Vacuum gases/vapours High pressure gases/vapours Shell-side liquids • Falls within accepted range 39 TP - heat exchanger design. ppt 10 to 30 m/s 50 to 70 m/s 5 to 10 m/s 0. 3 to 1. 0 m/s
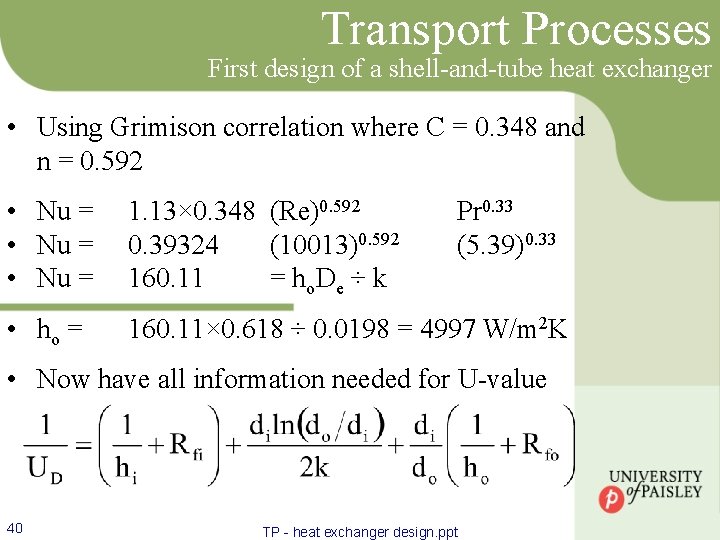
Transport Processes First design of a shell-and-tube heat exchanger • Using Grimison correlation where C = 0. 348 and n = 0. 592 • Nu = 1. 13× 0. 348 (Re)0. 592 0. 39324 (10013)0. 592 160. 11 = ho. De ÷ k • ho = 160. 11× 0. 618 ÷ 0. 0198 = 4997 W/m 2 K Pr 0. 33 (5. 39)0. 33 • Now have all information needed for U-value 40 TP - heat exchanger design. ppt
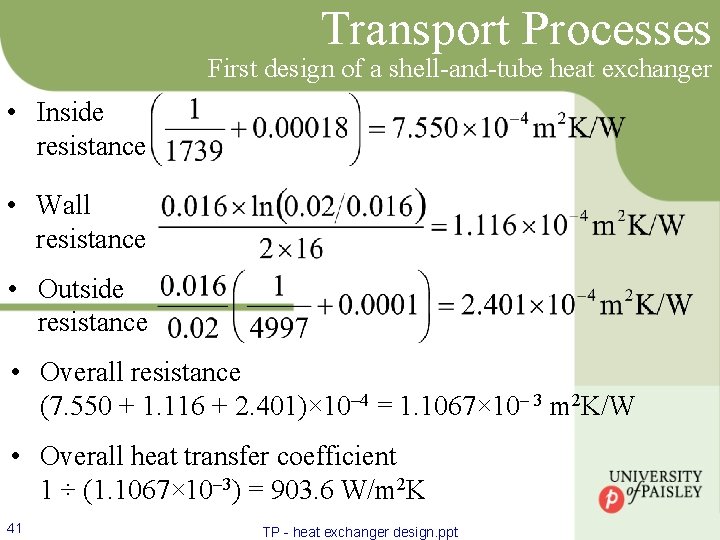
Transport Processes First design of a shell-and-tube heat exchanger • Inside resistance • Wall resistance • Outside resistance • Overall resistance (7. 550 + 1. 116 + 2. 401)× 10– 4 = 1. 1067× 10– 3 m 2 K/W • Overall heat transfer coefficient 1 ÷ (1. 1067× 10– 3) = 903. 6 W/m 2 K 41 TP - heat exchanger design. ppt
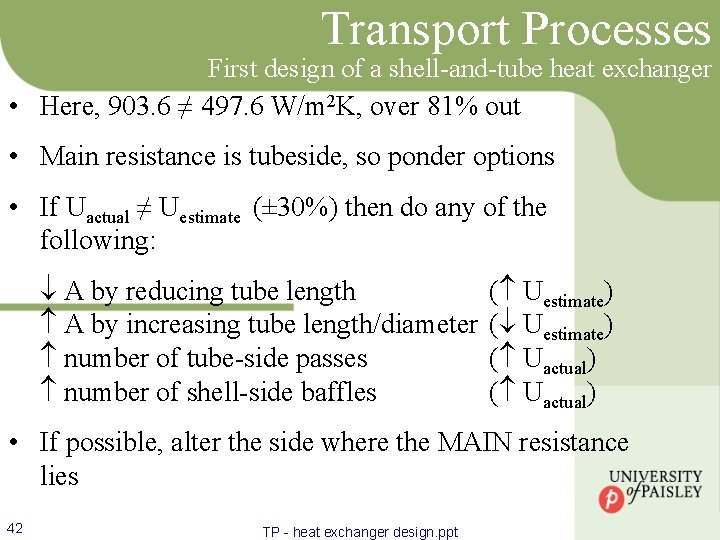
Transport Processes First design of a shell-and-tube heat exchanger • Here, 903. 6 ≠ 497. 6 W/m 2 K, over 81% out • Main resistance is tubeside, so ponder options • If Uactual ≠ Uestimate (± 30%) then do any of the following: A by reducing tube length A by increasing tube length/diameter number of tube-side passes number of shell-side baffles ( Uestimate) ( Uactual) • If possible, alter the side where the MAIN resistance lies 42 TP - heat exchanger design. ppt
Heat transfer overview
Tube pitch formula
The overall heat transfer coefficient
Seven fundamental
Kanolu
In shell and tube surface condenser
Convective heat transfer coefficient equation
Introduction to convection
Radiation heat transfer coefficient
Shell
Identify the following processes of transfer of heat.
Concurrent processes are processes that
The overall reaction in a commercial heat pack
The unit of mass transfer coefficient is
Ficks law
Transfer coefficient finance
Primary and secondary transport
Active transport
Passive transport vs active transport venn diagram
Active vs passive transport venn diagram
Pinocytosis vs phagocytosis
Primary active transport vs secondary active transport
Bioflix activity membrane transport active transport
Active and passive transport
Bioflix activity membrane transport active transport
A gas cylinder and piston are covered with heavy insulation
Principles of reliable data transfer in transport layer
Reliable data transfer in transport layer
Reliable data transfer in transport layer
Heat transport
Disturbance that transfers energy
Heat transfer of metals
How does heat energy flow
What is heat
Diffusion equation heat transfer
Heat transfer jeopardy
Four containers were filled with warm water
Principle of kettle evaporator
Heat transfer by conduction gizmo
Ntu in heat exchanger
Ntu heat transfer
We transfer
Examples of radiation