Cell MembranePlasma Membrane plasma membrane is the boundary
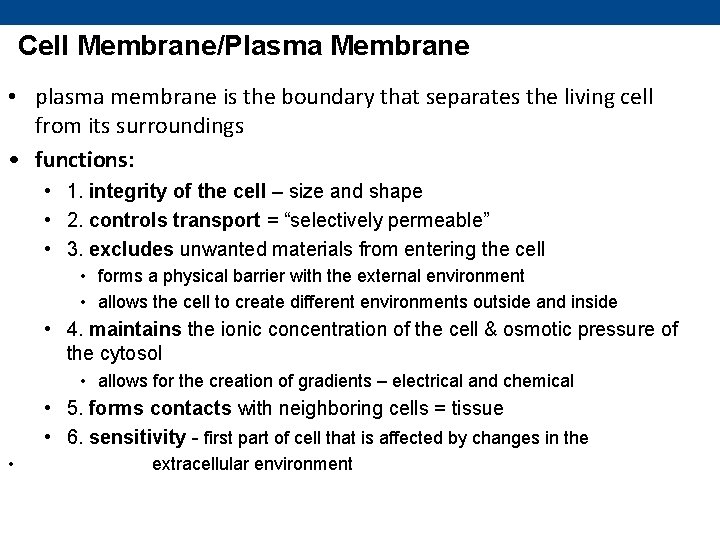
Cell Membrane/Plasma Membrane • plasma membrane is the boundary that separates the living cell from its surroundings • functions: • 1. integrity of the cell – size and shape • 2. controls transport = “selectively permeable” • 3. excludes unwanted materials from entering the cell • forms a physical barrier with the external environment • allows the cell to create different environments outside and inside • 4. maintains the ionic concentration of the cell & osmotic pressure of the cytosol • allows for the creation of gradients – electrical and chemical • 5. forms contacts with neighboring cells = tissue • 6. sensitivity - first part of cell that is affected by changes in the • extracellular environment
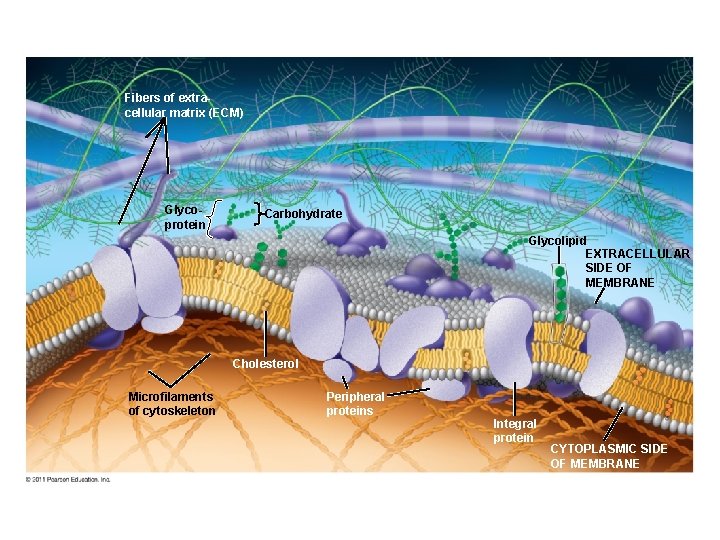
Fibers of extracellular matrix (ECM) Glycoprotein Carbohydrate Glycolipid EXTRACELLULAR SIDE OF MEMBRANE Cholesterol Microfilaments of cytoskeleton Peripheral proteins Integral protein CYTOPLASMIC SIDE OF MEMBRANE
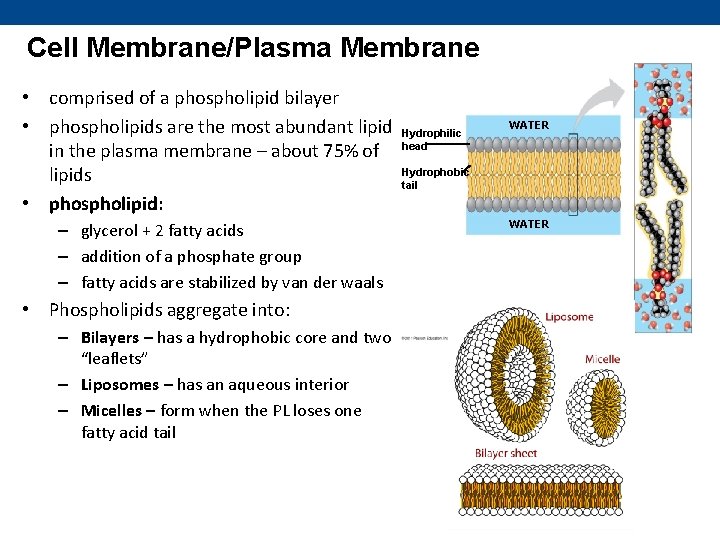
Cell Membrane/Plasma Membrane • comprised of a phospholipid bilayer • phospholipids are the most abundant lipid in the plasma membrane – about 75% of lipids • phospholipid: – glycerol + 2 fatty acids – addition of a phosphate group – fatty acids are stabilized by van der waals • Phospholipids aggregate into: – Bilayers – has a hydrophobic core and two “leaflets” – Liposomes – has an aqueous interior – Micelles – form when the PL loses one fatty acid tail Hydrophilic head WATER Hydrophobic tail WATER
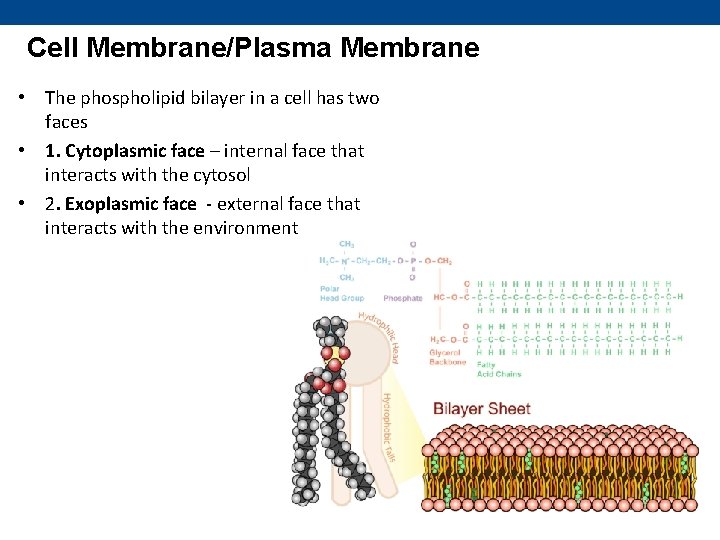
Cell Membrane/Plasma Membrane • The phospholipid bilayer in a cell has two faces • 1. Cytoplasmic face – internal face that interacts with the cytosol • 2. Exoplasmic face - external face that interacts with the environment
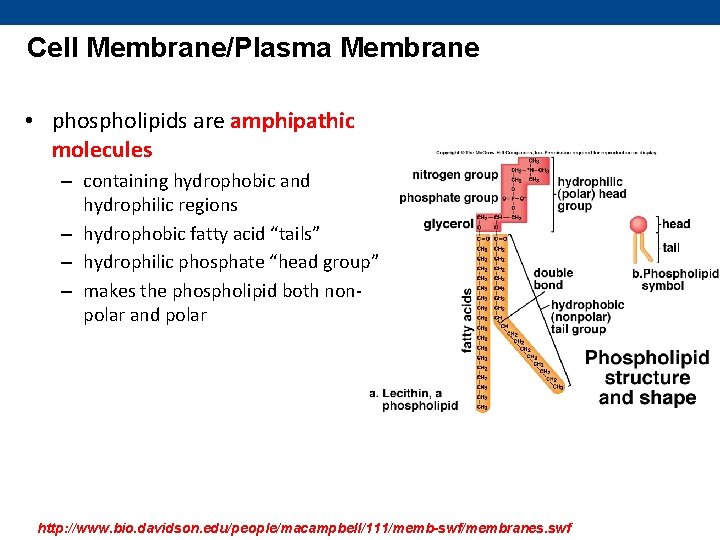
Cell Membrane/Plasma Membrane • phospholipids are amphipathic molecules – containing hydrophobic and hydrophilic regions – hydrophobic fatty acid “tails” – hydrophilic phosphate “head group” – makes the phospholipid both nonpolar and polar http: //www. bio. davidson. edu/people/macampbell/111/memb-swf/membranes. swf
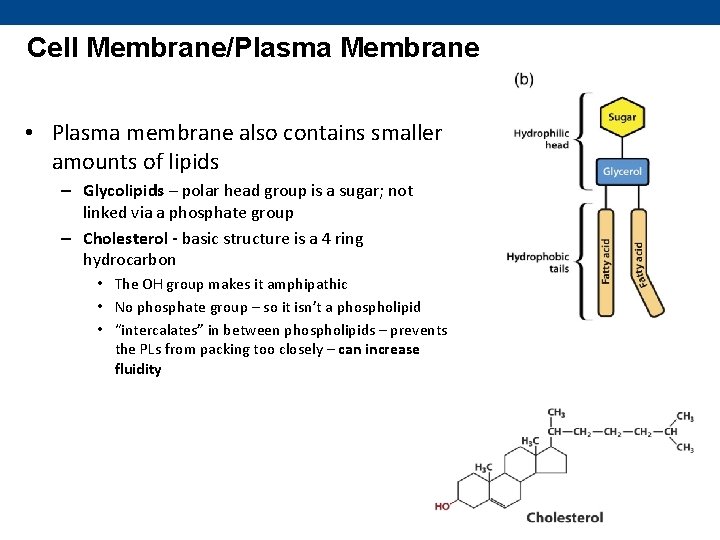
Cell Membrane/Plasma Membrane • Plasma membrane also contains smaller amounts of lipids – Glycolipids – polar head group is a sugar; not linked via a phosphate group – Cholesterol - basic structure is a 4 ring hydrocarbon • The OH group makes it amphipathic • No phosphate group – so it isn’t a phospholipid • “intercalates” in between phospholipids – prevents the PLs from packing too closely – can increase fluidity
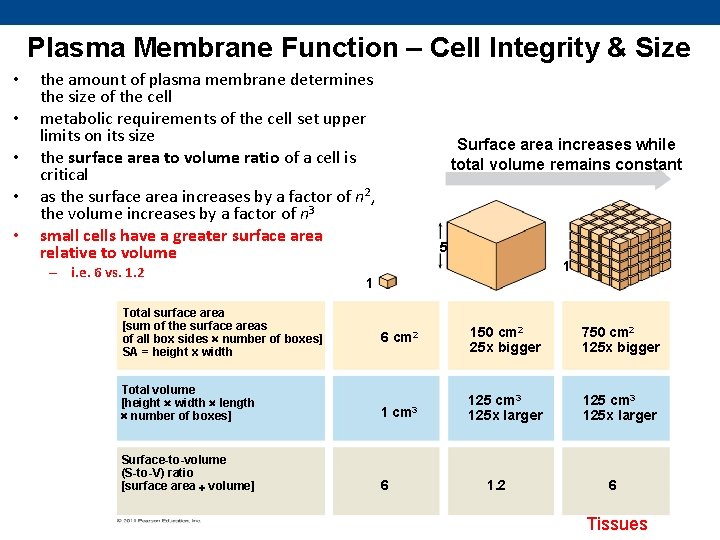
Plasma Membrane Function – Cell Integrity & Size • • • the amount of plasma membrane determines the size of the cell metabolic requirements of the cell set upper limits on its size the surface area to volume ratio of a cell is critical as the surface area increases by a factor of n 2, the volume increases by a factor of n 3 small cells have a greater surface area relative to volume – i. e. 6 vs. 1. 2 Total surface area [sum of the surface areas of all box sides number of boxes] SA = height x width Surface area increases while total volume remains constant 5 1 1 6 cm 2 150 cm 2 25 x bigger 750 cm 2 125 x bigger cm 3 125 x larger 125 cm 3 125 x larger Total volume [height width length number of boxes] 1 Surface-to-volume (S-to-V) ratio [surface area volume] 6 1. 2 6 Tissues
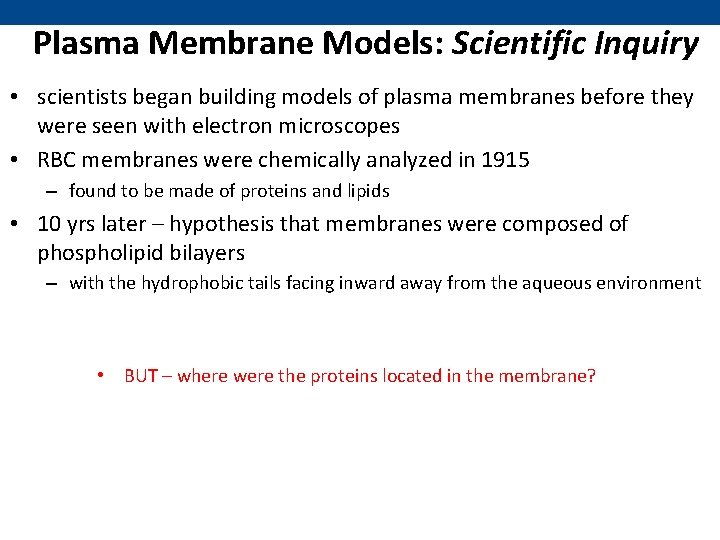
Plasma Membrane Models: Scientific Inquiry • scientists began building models of plasma membranes before they were seen with electron microscopes • RBC membranes were chemically analyzed in 1915 – found to be made of proteins and lipids • 10 yrs later – hypothesis that membranes were composed of phospholipid bilayers – with the hydrophobic tails facing inward away from the aqueous environment • BUT – where were the proteins located in the membrane?
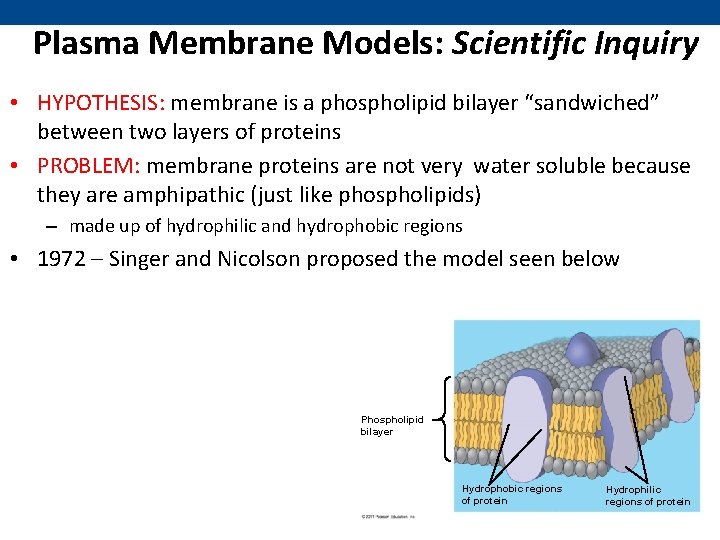
Plasma Membrane Models: Scientific Inquiry • HYPOTHESIS: membrane is a phospholipid bilayer “sandwiched” between two layers of proteins • PROBLEM: membrane proteins are not very water soluble because they are amphipathic (just like phospholipids) – made up of hydrophilic and hydrophobic regions • 1972 – Singer and Nicolson proposed the model seen below Phospholipid bilayer Hydrophobic regions of protein Hydrophilic regions of protein
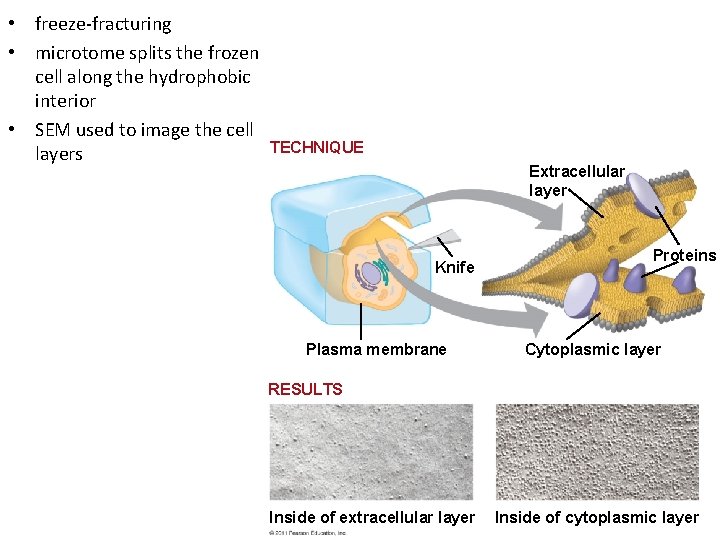
• freeze-fracturing • microtome splits the frozen cell along the hydrophobic interior • SEM used to image the cell layers TECHNIQUE Extracellular layer Knife Plasma membrane Proteins Cytoplasmic layer RESULTS Inside of extracellular layer Inside of cytoplasmic layer
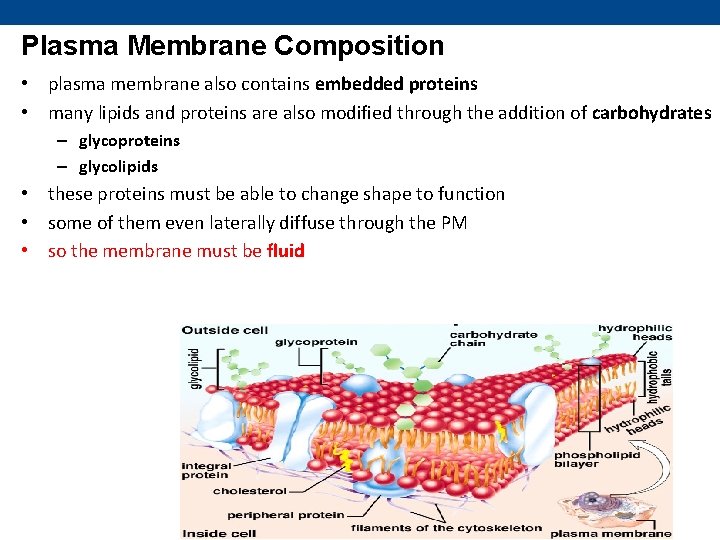
Plasma Membrane Composition • plasma membrane also contains embedded proteins • many lipids and proteins are also modified through the addition of carbohydrates – glycoproteins – glycolipids • these proteins must be able to change shape to function • some of them even laterally diffuse through the PM • so the membrane must be fluid

Plasma Membrane Fluidity • fluid mosaic model states that a membrane is a fluid structure with a “mosaic” of various proteins embedded in it • membrane fluidity is due to several factors – – temperature - lipids move around more with increased temp lipid packing – lipids with shorter fatty acid tails are less stiff saturation of fatty acids – more C=C bonds (more unsaturated) increase fluidity cholesterol – decreases fluidity at warmer temps; increases fluidity at lower temps Lateral movement occurs 107 times per second. Flip-flopping across the membrane is rare ( once per month).

Scientific Inquiry: Do membrane proteins move? • Frye and Eddin – fluorescently labelled membrane proteins of mouse and human cells and fused the cells • production of “hybrid” cells with a homogenous mixture of proteins proved that proteins do move within the plasma membrane RESULTS Membrane proteins Mouse cell Mixed proteins after 1 hour Human cell Hybrid cell
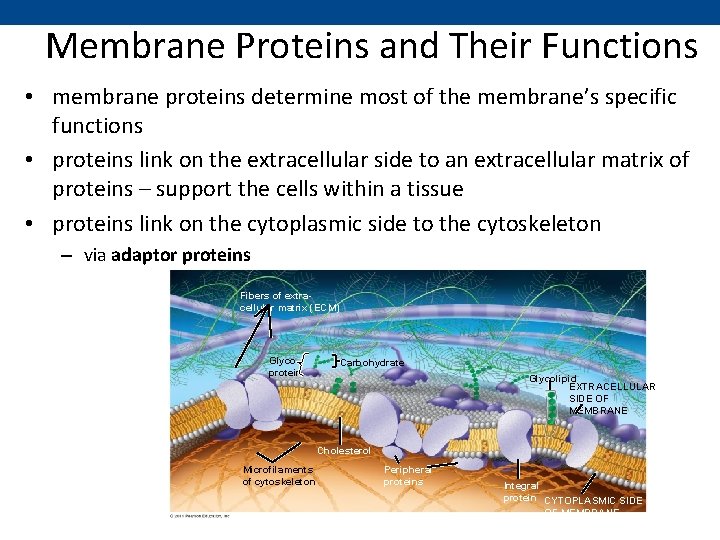
Membrane Proteins and Their Functions • membrane proteins determine most of the membrane’s specific functions • proteins link on the extracellular side to an extracellular matrix of proteins – support the cells within a tissue • proteins link on the cytoplasmic side to the cytoskeleton – via adaptor proteins Fibers of extracellular matrix (ECM) Glycoprotein Carbohydrate Glycolipid EXTRACELLULAR SIDE OF MEMBRANE Cholesterol Microfilaments of cytoskeleton Peripheral proteins Integral protein CYTOPLASMIC SIDE OF MEMBRANE
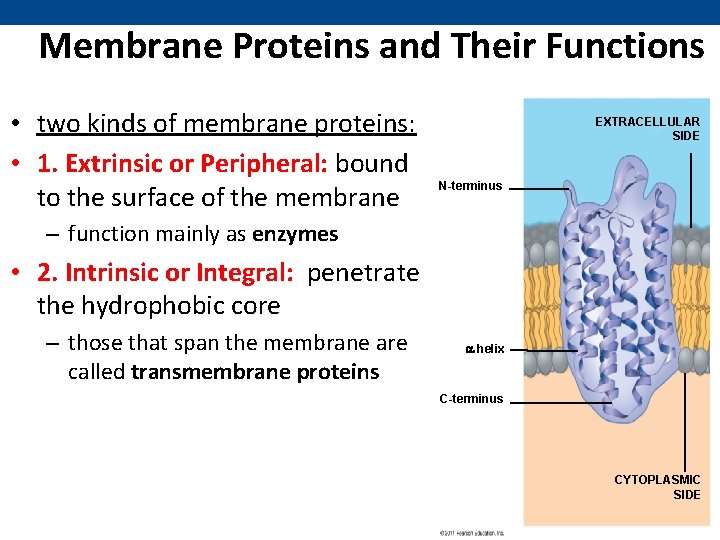
Membrane Proteins and Their Functions • two kinds of membrane proteins: • 1. Extrinsic or Peripheral: bound to the surface of the membrane EXTRACELLULAR SIDE N-terminus – function mainly as enzymes • 2. Intrinsic or Integral: penetrate the hydrophobic core – those that span the membrane are called transmembrane proteins helix C-terminus CYTOPLASMIC SIDE

• 6 major functions of integral membrane proteins: – 1. transport – channel proteins & transporters – 2. enzymatic activity – 3. signal transduction – receptor proteins – 4. cell-cell recognition – cell identity markers – 5. intercellular joining - linkers – 6. attachment- to the cytoskeleton and extracellular matrix (ECM) Enzymes Glycoprotein Enzymatic activity Cell-cell recognition Intercellular joining Attachment to the cytoskeleton and extracellular matrix (ECM)
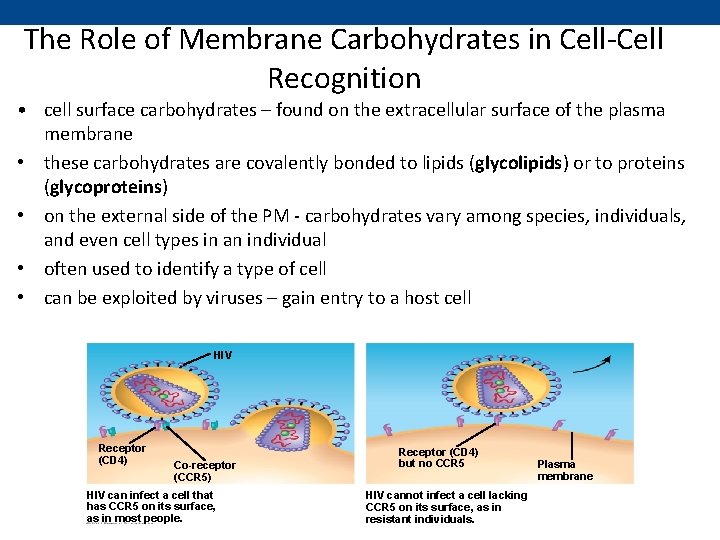
The Role of Membrane Carbohydrates in Cell-Cell Recognition • cell surface carbohydrates – found on the extracellular surface of the plasma membrane • these carbohydrates are covalently bonded to lipids (glycolipids) or to proteins (glycoproteins) • on the external side of the PM - carbohydrates vary among species, individuals, and even cell types in an individual • often used to identify a type of cell • can be exploited by viruses – gain entry to a host cell HIV Receptor (CD 4) Co-receptor (CCR 5) HIV can infect a cell that has CCR 5 on its surface, as in most people. Receptor (CD 4) but no CCR 5 HIV cannot infect a cell lacking CCR 5 on its surface, as in resistant individuals. Plasma membrane
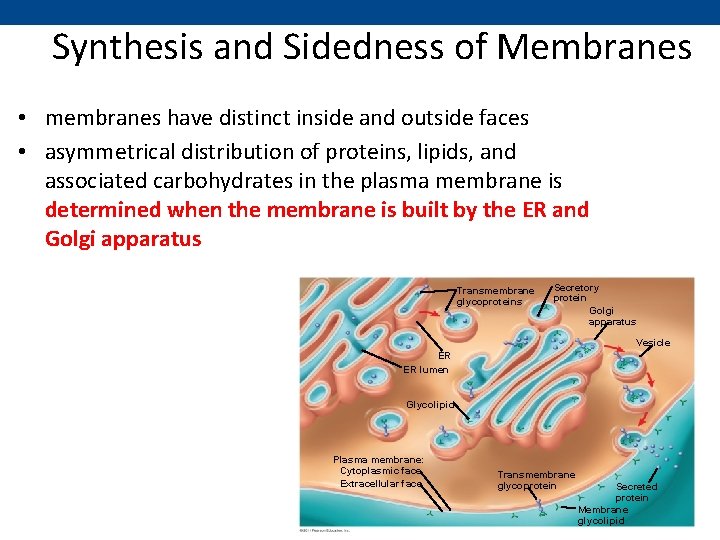
Synthesis and Sidedness of Membranes • membranes have distinct inside and outside faces • asymmetrical distribution of proteins, lipids, and associated carbohydrates in the plasma membrane is determined when the membrane is built by the ER and Golgi apparatus Transmembrane glycoproteins Secretory protein Golgi apparatus Vesicle ER ER lumen Glycolipid Plasma membrane: Cytoplasmic face Extracellular face Transmembrane glycoprotein Secreted protein Membrane glycolipid
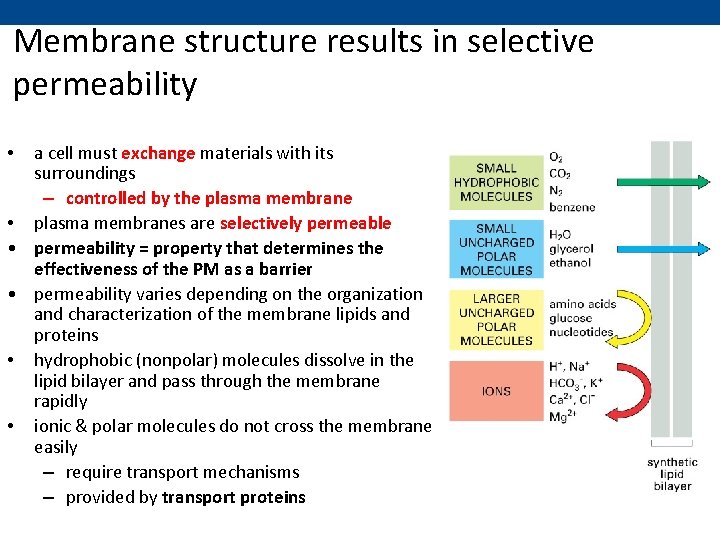
Membrane structure results in selective permeability a cell must exchange materials with its surroundings – controlled by the plasma membrane • plasma membranes are selectively permeable • permeability = property that determines the effectiveness of the PM as a barrier • permeability varies depending on the organization and characterization of the membrane lipids and proteins • hydrophobic (nonpolar) molecules dissolve in the lipid bilayer and pass through the membrane rapidly • ionic & polar molecules do not cross the membrane easily – require transport mechanisms – provided by transport proteins •
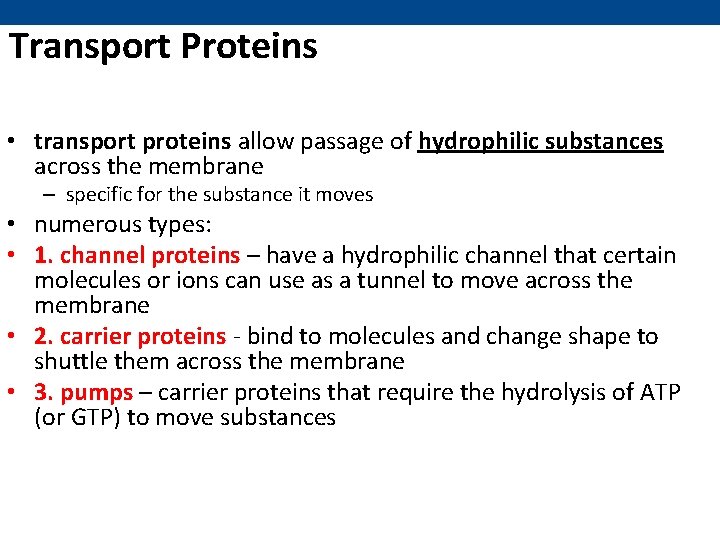
Transport Proteins • transport proteins allow passage of hydrophilic substances across the membrane – specific for the substance it moves • numerous types: • 1. channel proteins – have a hydrophilic channel that certain molecules or ions can use as a tunnel to move across the membrane • 2. carrier proteins - bind to molecules and change shape to shuttle them across the membrane • 3. pumps – carrier proteins that require the hydrolysis of ATP (or GTP) to move substances
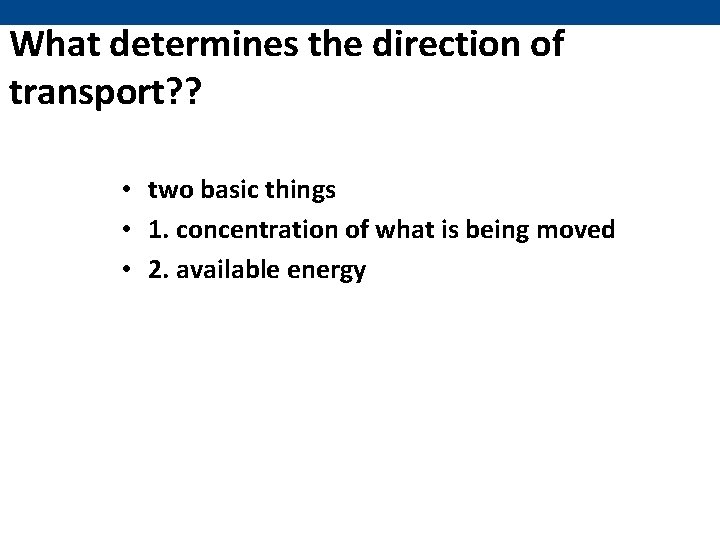
What determines the direction of transport? ? • two basic things • 1. concentration of what is being moved • 2. available energy
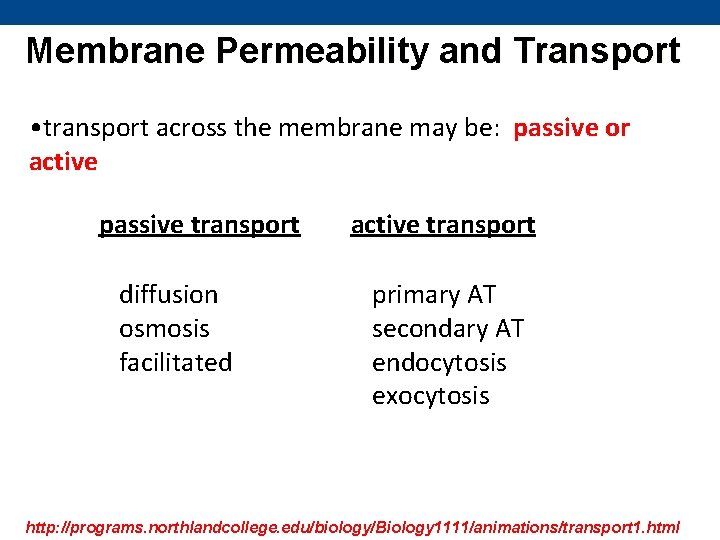
Membrane Permeability and Transport • transport across the membrane may be: passive or active passive transport diffusion osmosis facilitated active transport primary AT secondary AT endocytosis exocytosis http: //programs. northlandcollege. edu/biology/Biology 1111/animations/transport 1. html
![A. Diffusion = movement of materials from [high] to [low] -random movement, no energy A. Diffusion = movement of materials from [high] to [low] -random movement, no energy](http://slidetodoc.com/presentation_image_h/20ba28bc98b26761b6678e8e565c1c2b/image-23.jpg)
A. Diffusion = movement of materials from [high] to [low] -random movement, no energy needs to be synthesized -the movement is driven by the inherent kinetic energy of the particles moving down their concentration gradient -three ways to diffuse: 1. through the lipid bilayer: lipid soluble (non-polar), alcohol, gases, ammonia, fat-soluble vitamins 2. through a channel: charged ions or small polar molecules -some channels are “gated” – open and close 3. facilitated diffusion: larger, polar molecules too big for channels -uses a transporter protein
![B. Osmosis = diffusion of water from [high] to [low] OR movement of water B. Osmosis = diffusion of water from [high] to [low] OR movement of water](http://slidetodoc.com/presentation_image_h/20ba28bc98b26761b6678e8e565c1c2b/image-24.jpg)
B. Osmosis = diffusion of water from [high] to [low] OR movement of water from [low solute] to [high solute] -in osmosis – the membrane is permeable to water and NOT to the solutes -BUT it is the concentration of solutes that causes the water to move -the solutes are surround by a hydration shell of water molecules -this decreases the amount of free water molecules available to move Lower concentration of solute (sugar) Higher concentration of solute Sugar molecule H 2 O Selectively permeable membrane -so increased solute concentration decreases the concentration of free water molecules -water movement is affected by this drop in free water molecule concentration Osmosis Same concentration of solute
![B. Osmosis = diffusion of water from [high] to [low] OR movement of water B. Osmosis = diffusion of water from [high] to [low] OR movement of water](http://slidetodoc.com/presentation_image_h/20ba28bc98b26761b6678e8e565c1c2b/image-25.jpg)
B. Osmosis = diffusion of water from [high] to [low] OR movement of water from [low solute] to [high solute] -EXPERIMENT: U shaped tube divided by a membrane permeable to water only -increase the solute concentration in the right half of the tube -decrease free water concentration on the right side also -water rises on the side of the solutes – due to osmosis -counteract this rise in water by applying pressure = osmotic pressure (OP) -therefore increasing solute concentration increases osmotic pressure -water will move toward that area to decrease this OP
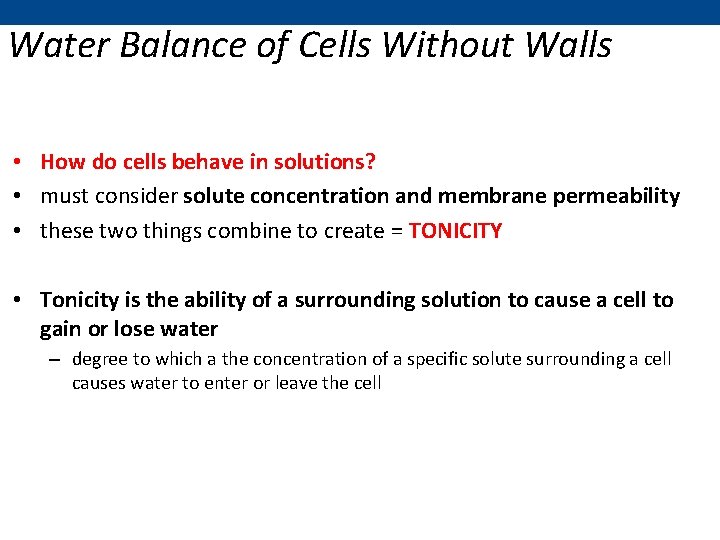
Water Balance of Cells Without Walls • How do cells behave in solutions? • must consider solute concentration and membrane permeability • these two things combine to create = TONICITY • Tonicity is the ability of a surrounding solution to cause a cell to gain or lose water – degree to which a the concentration of a specific solute surrounding a cell causes water to enter or leave the cell
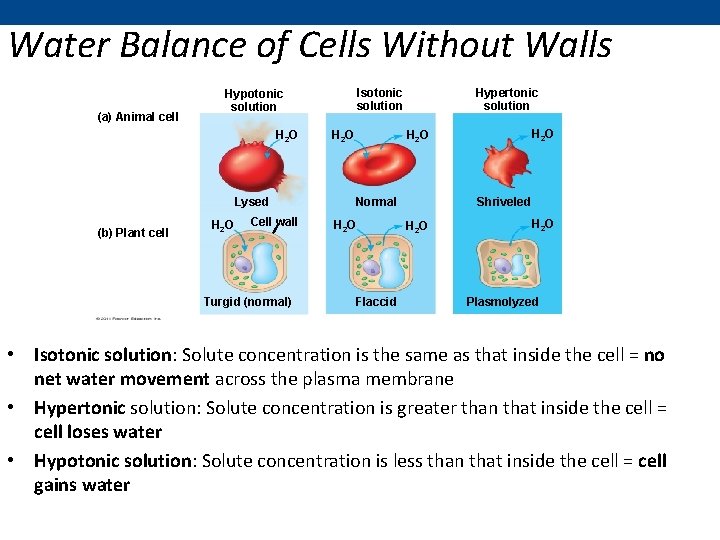
Water Balance of Cells Without Walls (a) Animal cell H 2 O Lysed (b) Plant cell Isotonic solution Hypotonic solution H 2 O Cell wall Turgid (normal) H 2 O Hypertonic solution H 2 O Normal H 2 O Flaccid Shriveled H 2 O Plasmolyzed Osmosis • Isotonic solution: Solute concentration is the same as that inside the cell = no net water movement across the plasma membrane • Hypertonic solution: Solute concentration is greater than that inside the cell = cell loses water • Hypotonic solution: Solute concentration is less than that inside the cell = cell gains water
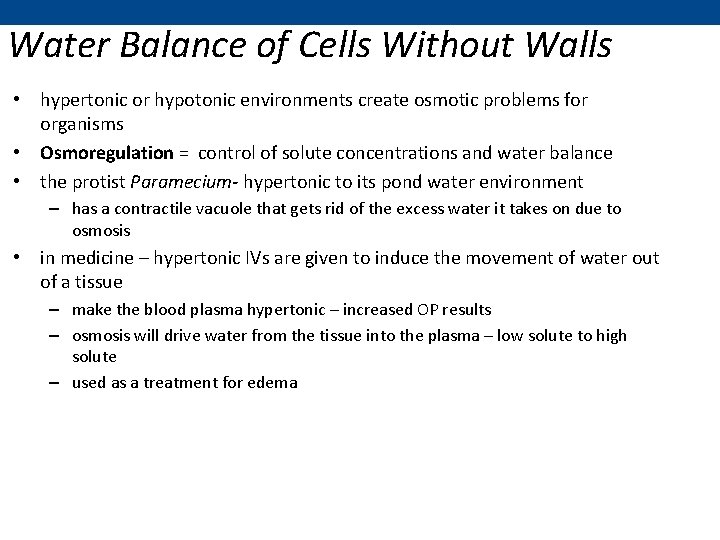
Water Balance of Cells Without Walls • hypertonic or hypotonic environments create osmotic problems for organisms • Osmoregulation = control of solute concentrations and water balance • the protist Paramecium- hypertonic to its pond water environment – has a contractile vacuole that gets rid of the excess water it takes on due to osmosis • in medicine – hypertonic IVs are given to induce the movement of water out of a tissue – make the blood plasma hypertonic – increased OP results – osmosis will drive water from the tissue into the plasma – low solute to high solute – used as a treatment for edema
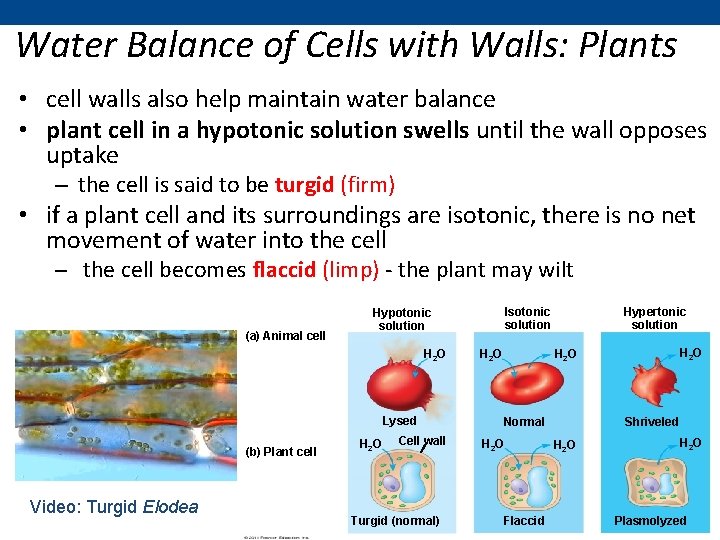
Water Balance of Cells with Walls: Plants • cell walls also help maintain water balance • plant cell in a hypotonic solution swells until the wall opposes uptake – the cell is said to be turgid (firm) • if a plant cell and its surroundings are isotonic, there is no net movement of water into the cell – the cell becomes flaccid (limp) - the plant may wilt (a) Animal cell H 2 O Lysed (b) Plant cell Video: Turgid Elodea Isotonic solution Hypotonic solution H 2 O Cell wall Turgid (normal) H 2 O Hypertonic solution H 2 O Normal H 2 O Flaccid Shriveled H 2 O Plasmolyzed
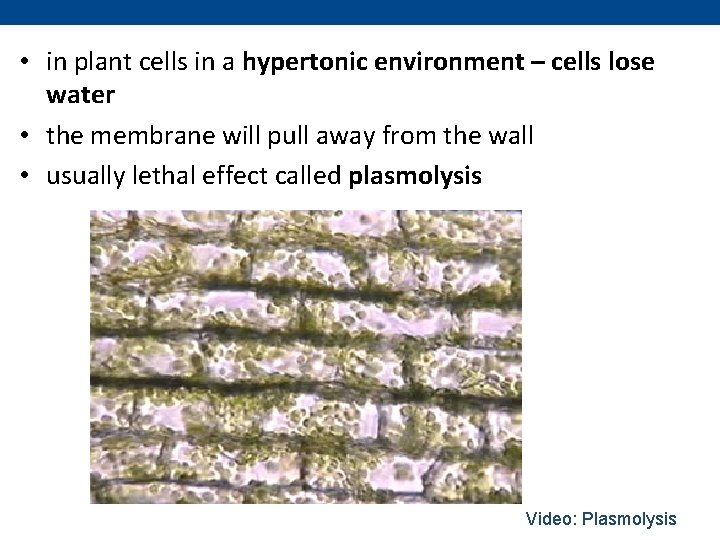
• in plant cells in a hypertonic environment – cells lose water • the membrane will pull away from the wall • usually lethal effect called plasmolysis Video: Plasmolysis
![Facilitated Transport/Diffusion • movement of a molecule from [high] to [low] aided by a Facilitated Transport/Diffusion • movement of a molecule from [high] to [low] aided by a](http://slidetodoc.com/presentation_image_h/20ba28bc98b26761b6678e8e565c1c2b/image-31.jpg)
Facilitated Transport/Diffusion • movement of a molecule from [high] to [low] aided by a protein - the transport protein is either: 1. through a channel protein e. g aquaporins- for facilitated diffusion of water e. g. ion channels that open or close in response to a stimulus (gated channels) 2. through a carrier protein EXTRACELLULAR FLUID Channel protein CYTOPLASM Solute

Carrier proteins and Facilitated Diffusion -solute binds to the carrier protein located on the plasma membrane -transported across the membrane by the carrier protein’s change in shape -no energy required – still moving high to low concentration -but there is a limit to the amount of facilitated diffusion the cells can undergo - has to do with the # of carrier proteins on the PM EXTRACELLULAR FLUID Carrier protein CYTOPLASM (b) A carrier protein Solute

Active transport uses energy to move solutes against their gradients • some transport proteins can move solutes against their concentration gradients • Active transport moves substances against their concentration gradients – requires energy, usually in the form of ATP – is performed by specific proteins embedded in the membranes called pumps • two kinds of active transport: – Primary – Secondary
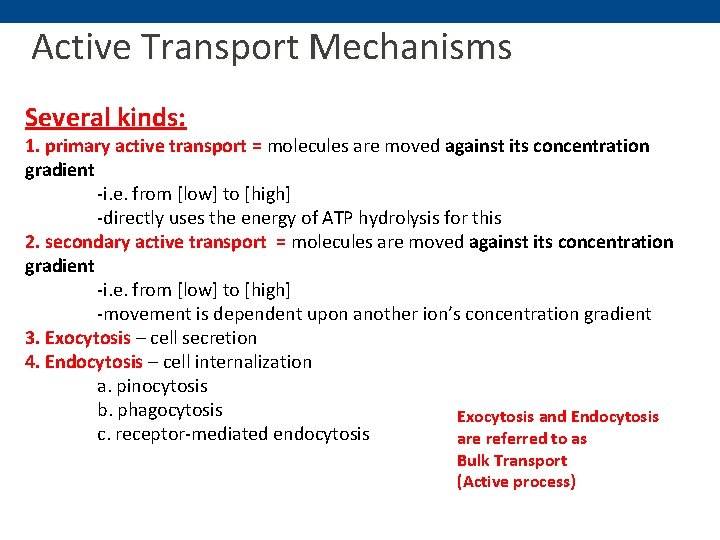
Active Transport Mechanisms Several kinds: 1. primary active transport = molecules are moved against its concentration gradient -i. e. from [low] to [high] -directly uses the energy of ATP hydrolysis for this 2. secondary active transport = molecules are moved against its concentration gradient -i. e. from [low] to [high] -movement is dependent upon another ion’s concentration gradient 3. Exocytosis – cell secretion 4. Endocytosis – cell internalization a. pinocytosis b. phagocytosis Exocytosis and Endocytosis c. receptor-mediated endocytosis are referred to as Bulk Transport (Active process)
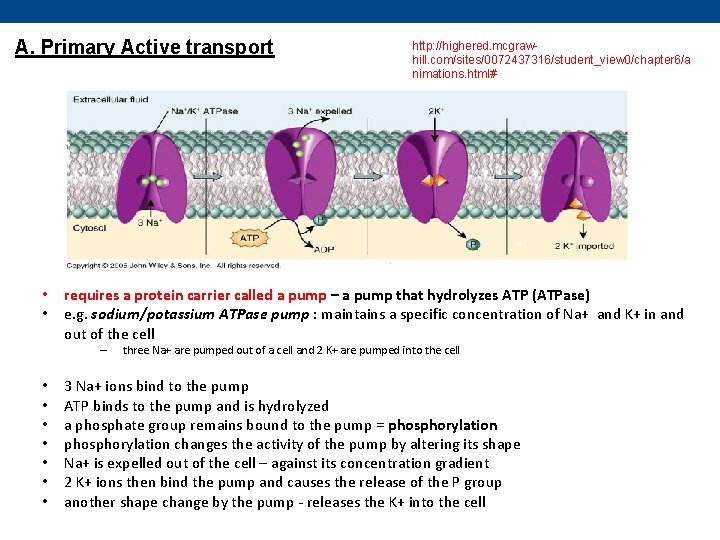
A. Primary Active transport • • requires a protein carrier called a pump – a pump that hydrolyzes ATP (ATPase) e. g. sodium/potassium ATPase pump : maintains a specific concentration of Na+ and K+ in and out of the cell – • • http: //highered. mcgrawhill. com/sites/0072437316/student_view 0/chapter 6/a nimations. html# three Na+ are pumped out of a cell and 2 K+ are pumped into the cell 3 Na+ ions bind to the pump ATP binds to the pump and is hydrolyzed a phosphate group remains bound to the pump = phosphorylation changes the activity of the pump by altering its shape Na+ is expelled out of the cell – against its concentration gradient 2 K+ ions then bind the pump and causes the release of the P group another shape change by the pump - releases the K+ into the cell
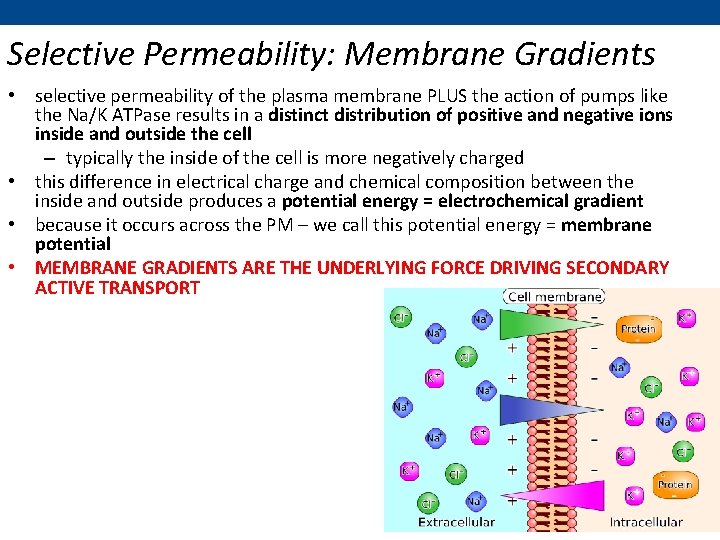
Selective Permeability: Membrane Gradients • selective permeability of the plasma membrane PLUS the action of pumps like the Na/K ATPase results in a distinct distribution of positive and negative ions inside and outside the cell – typically the inside of the cell is more negatively charged • this difference in electrical charge and chemical composition between the inside and outside produces a potential energy = electrochemical gradient • because it occurs across the PM – we call this potential energy = membrane potential • MEMBRANE GRADIENTS ARE THE UNDERLYING FORCE DRIVING SECONDARY ACTIVE TRANSPORT
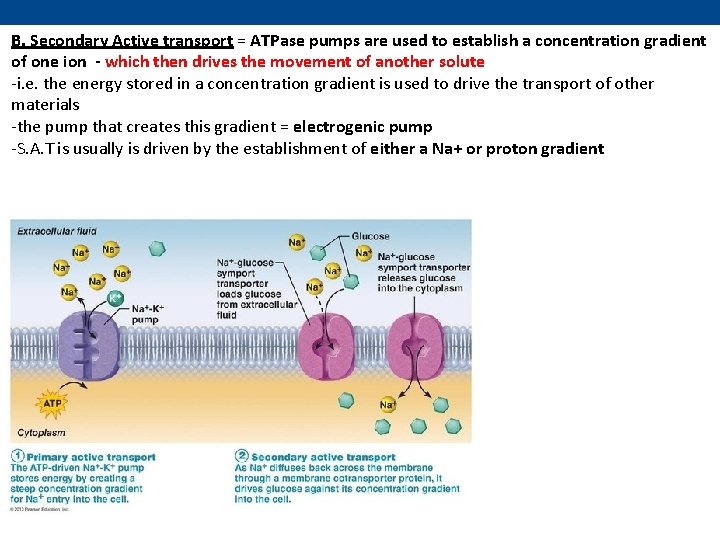
B. Secondary Active transport = ATPase pumps are used to establish a concentration gradient of one ion - which then drives the movement of another solute -i. e. the energy stored in a concentration gradient is used to drive the transport of other materials -the pump that creates this gradient = electrogenic pump -S. A. T is usually is driven by the establishment of either a Na+ or proton gradient
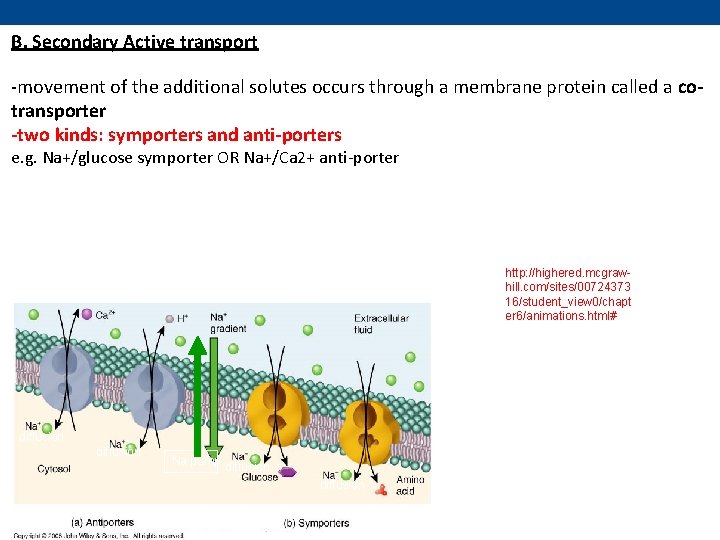
B. Secondary Active transport -movement of the additional solutes occurs through a membrane protein called a cotransporter -two kinds: symporters and anti-porters e. g. Na+/glucose symporter OR Na+/Ca 2+ anti-porter http: //highered. mcgrawhill. com/sites/00724373 16/student_view 0/chapt er 6/animations. html# diffusion Na pump diffusion
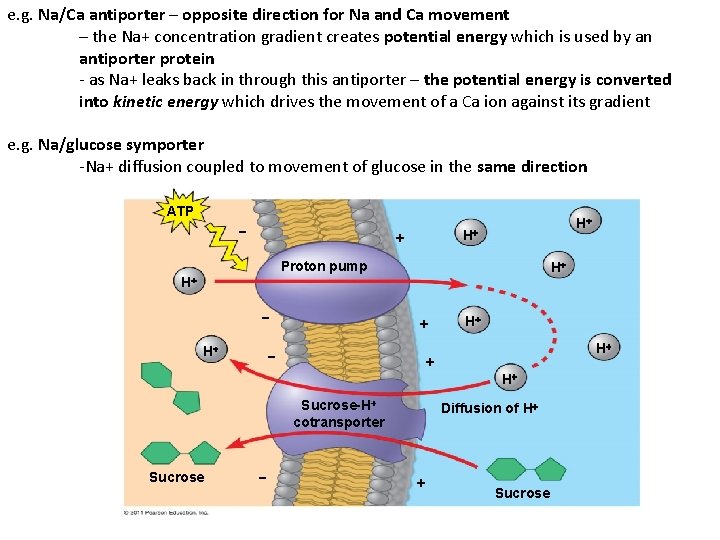
e. g. Na/Ca antiporter – opposite direction for Na and Ca movement – the Na+ concentration gradient creates potential energy which is used by an antiporter protein - as Na+ leaks back in through this antiporter – the potential energy is converted into kinetic energy which drives the movement of a Ca ion against its gradient e. g. Na/glucose symporter -Na+ diffusion coupled to movement of glucose in the same direction ATP H H Proton pump H H H H H Sucrose-H cotransporter Sucrose Diffusion of H Sucrose
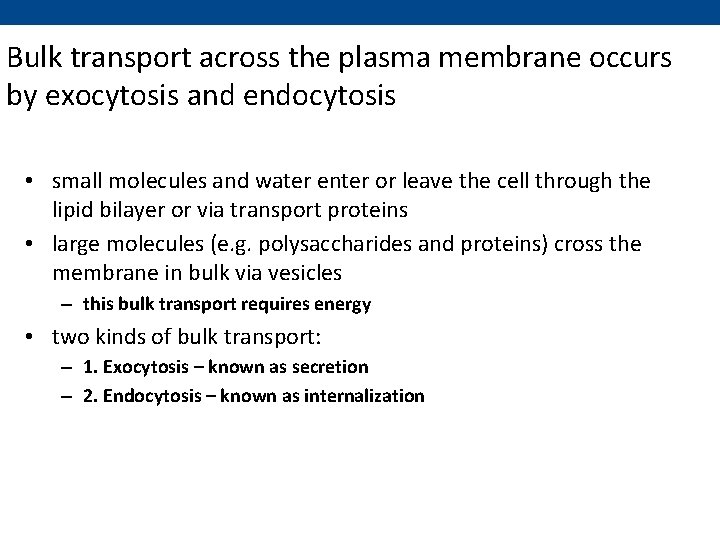
Bulk transport across the plasma membrane occurs by exocytosis and endocytosis • small molecules and water enter or leave the cell through the lipid bilayer or via transport proteins • large molecules (e. g. polysaccharides and proteins) cross the membrane in bulk via vesicles – this bulk transport requires energy • two kinds of bulk transport: – 1. Exocytosis – known as secretion – 2. Endocytosis – known as internalization
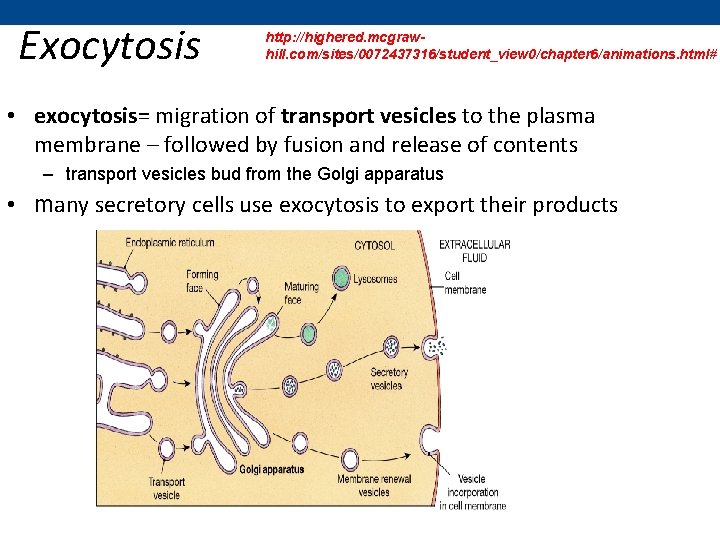
Exocytosis http: //highered. mcgrawhill. com/sites/0072437316/student_view 0/chapter 6/animations. html# • exocytosis= migration of transport vesicles to the plasma membrane – followed by fusion and release of contents – transport vesicles bud from the Golgi apparatus • many secretory cells use exocytosis to export their products
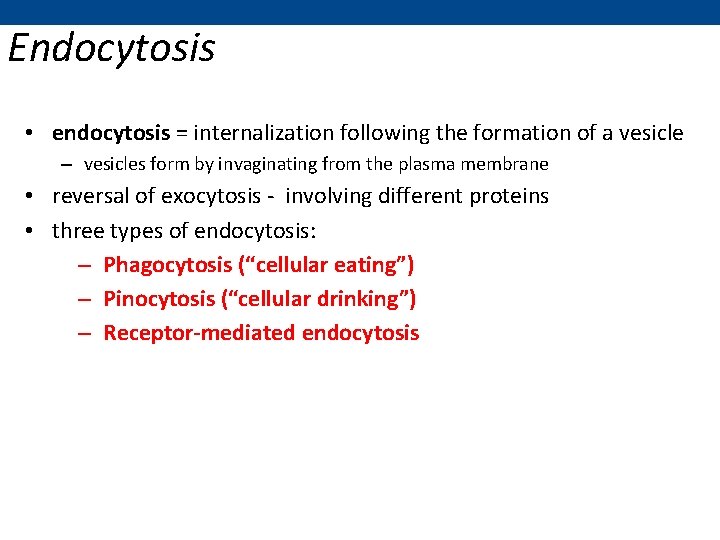
Endocytosis • endocytosis = internalization following the formation of a vesicle – vesicles form by invaginating from the plasma membrane • reversal of exocytosis - involving different proteins • three types of endocytosis: – Phagocytosis (“cellular eating”) – Pinocytosis (“cellular drinking”) – Receptor-mediated endocytosis
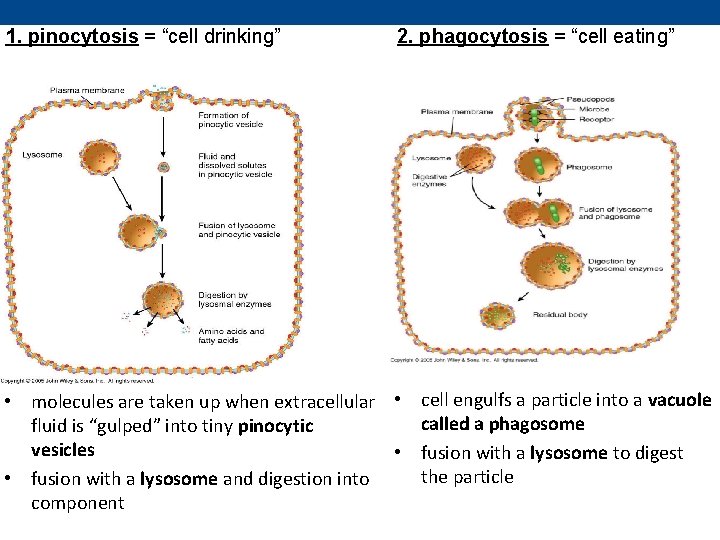
1. pinocytosis = “cell drinking” 2. phagocytosis = “cell eating” • molecules are taken up when extracellular • cell engulfs a particle into a vacuole called a phagosome fluid is “gulped” into tiny pinocytic vesicles • fusion with a lysosome to digest the particle • fusion with a lysosome and digestion into component
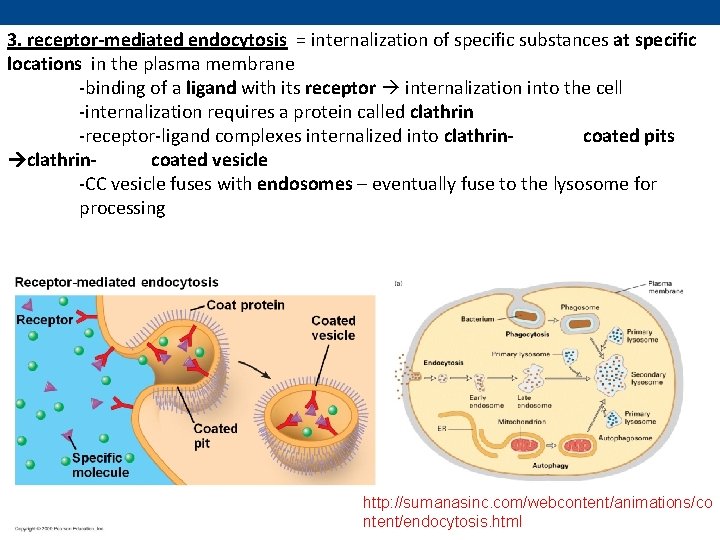
3. receptor-mediated endocytosis = internalization of specific substances at specific locations in the plasma membrane -binding of a ligand with its receptor internalization into the cell -internalization requires a protein called clathrin -receptor-ligand complexes internalized into clathrincoated pits clathrincoated vesicle -CC vesicle fuses with endosomes – eventually fuse to the lysosome for processing http: //sumanasinc. com/webcontent/animations/co ntent/endocytosis. html
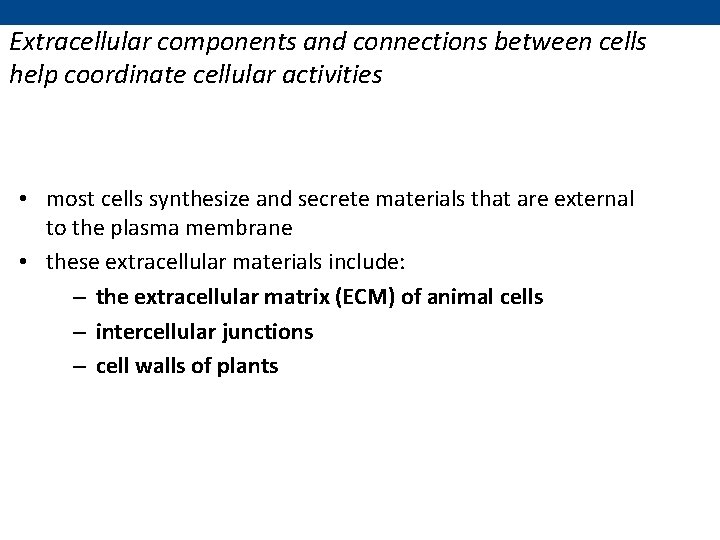
Extracellular components and connections between cells help coordinate cellular activities • most cells synthesize and secrete materials that are external to the plasma membrane • these extracellular materials include: – the extracellular matrix (ECM) of animal cells – intercellular junctions – cell walls of plants
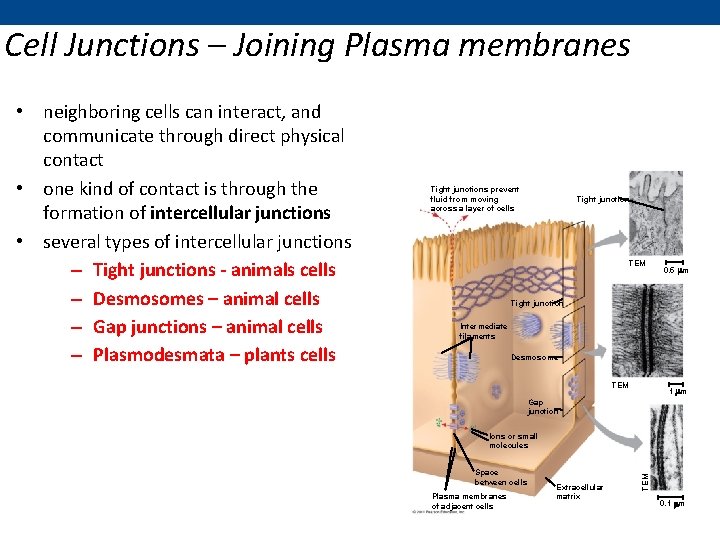
Cell Junctions – Joining Plasma membranes Tight junctions prevent fluid from moving across a layer of cells Tight junction TEM 0. 5 m Tight junction Intermediate filaments Desmosome TEM 1 m Gap junction Ions or small molecules Space between cells Plasma membranes of adjacent cells Extracellular matrix TEM • neighboring cells can interact, and communicate through direct physical contact • one kind of contact is through the formation of intercellular junctions • several types of intercellular junctions – Tight junctions - animals cells – Desmosomes – animal cells – Gap junctions – animal cells – Plasmodesmata – plants cells 0. 1 m
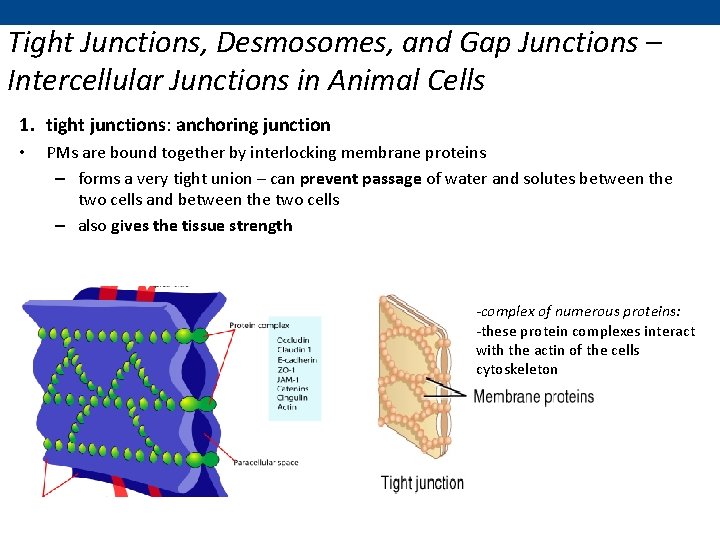
Tight Junctions, Desmosomes, and Gap Junctions – Intercellular Junctions in Animal Cells 1. tight junctions: anchoring junction • PMs are bound together by interlocking membrane proteins – forms a very tight union – can prevent passage of water and solutes between the two cells and between the two cells – also gives the tissue strength -complex of numerous proteins: -these protein complexes interact with the actin of the cells cytoskeleton
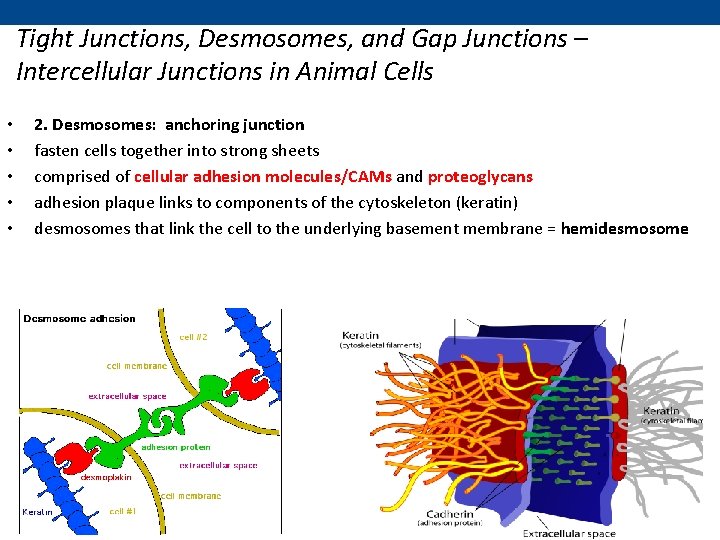
Tight Junctions, Desmosomes, and Gap Junctions – Intercellular Junctions in Animal Cells • • • 2. Desmosomes: anchoring junction fasten cells together into strong sheets comprised of cellular adhesion molecules/CAMs and proteoglycans adhesion plaque links to components of the cytoskeleton (keratin) desmosomes that link the cell to the underlying basement membrane = hemidesmosome
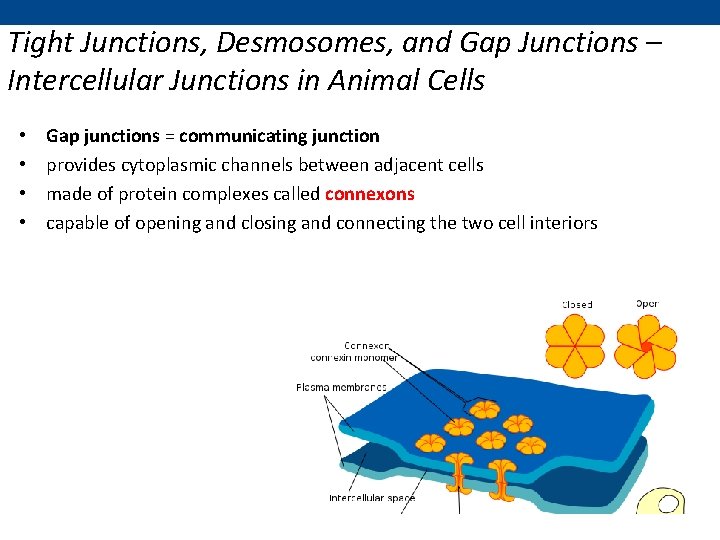
Tight Junctions, Desmosomes, and Gap Junctions – Intercellular Junctions in Animal Cells • • Gap junctions = communicating junction provides cytoplasmic channels between adjacent cells made of protein complexes called connexons capable of opening and closing and connecting the two cell interiors
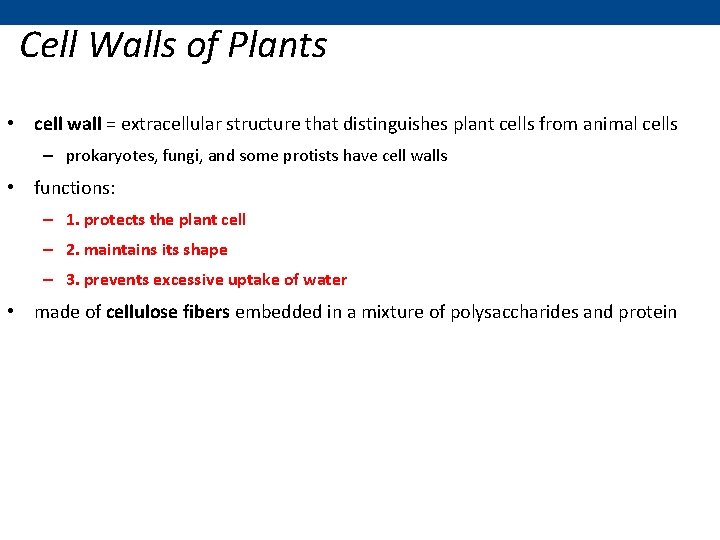
Cell Walls of Plants • cell wall = extracellular structure that distinguishes plant cells from animal cells – prokaryotes, fungi, and some protists have cell walls • functions: – 1. protects the plant cell – 2. maintains its shape – 3. prevents excessive uptake of water • made of cellulose fibers embedded in a mixture of polysaccharides and protein
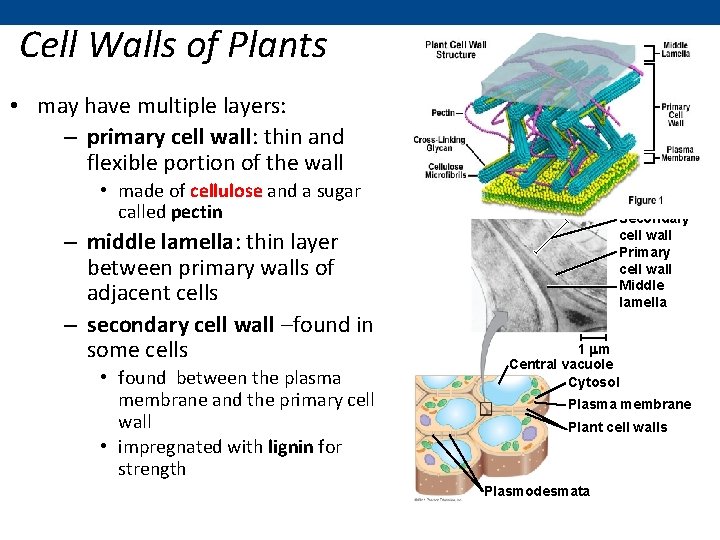
Cell Walls of Plants • may have multiple layers: – primary cell wall: thin and flexible portion of the wall • made of cellulose and a sugar called pectin – middle lamella: thin layer between primary walls of adjacent cells – secondary cell wall –found in some cells • found between the plasma membrane and the primary cell wall • impregnated with lignin for strength Secondary cell wall Primary cell wall Middle lamella 1 m Central vacuole Cytosol Plasma membrane Plant cell walls Plasmodesmata
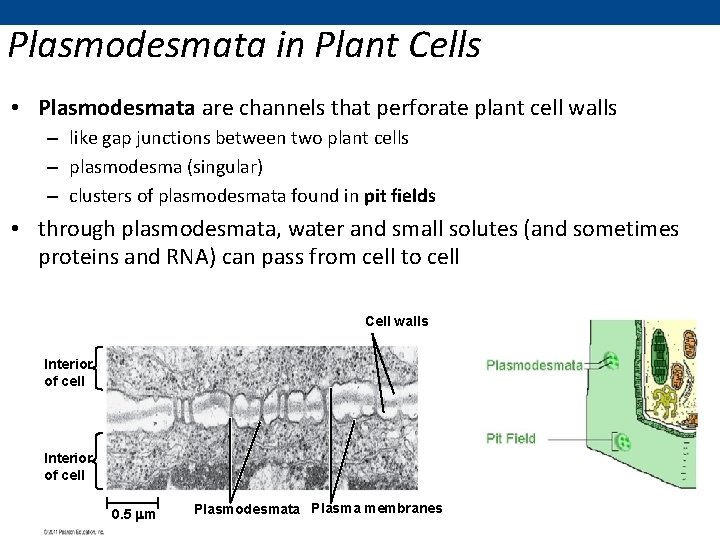
Plasmodesmata in Plant Cells • Plasmodesmata are channels that perforate plant cell walls – like gap junctions between two plant cells – plasmodesma (singular) – clusters of plasmodesmata found in pit fields • through plasmodesmata, water and small solutes (and sometimes proteins and RNA) can pass from cell to cell Cell walls Interior of cell 0. 5 m Plasmodesmata Plasma membranes
- Slides: 52