The LHC collider I Rdiger Schmidt CERN Klausurtagung
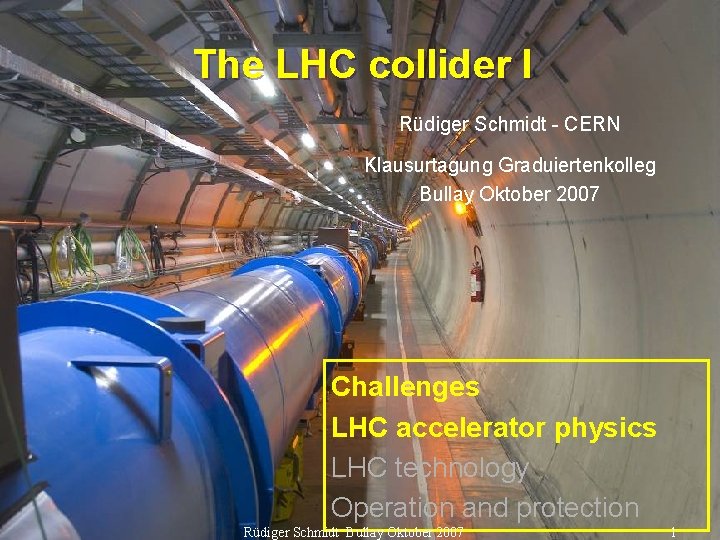
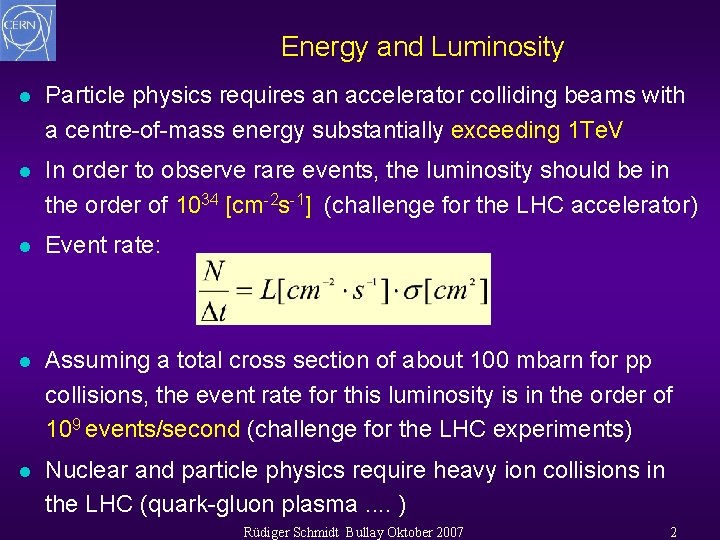
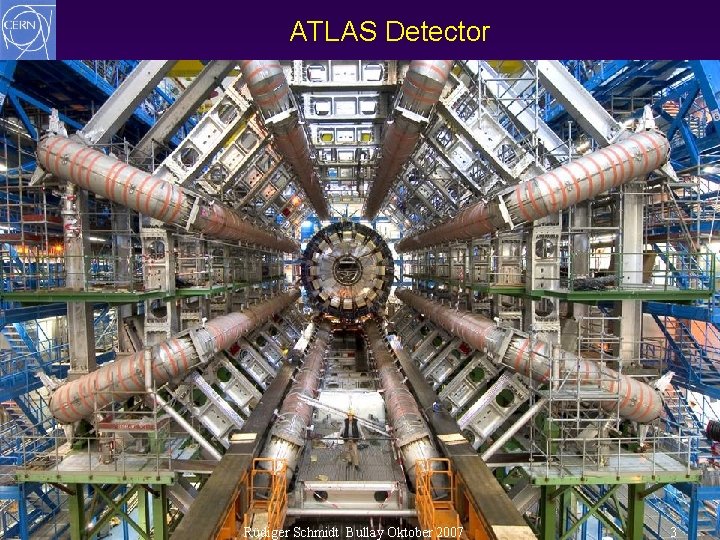
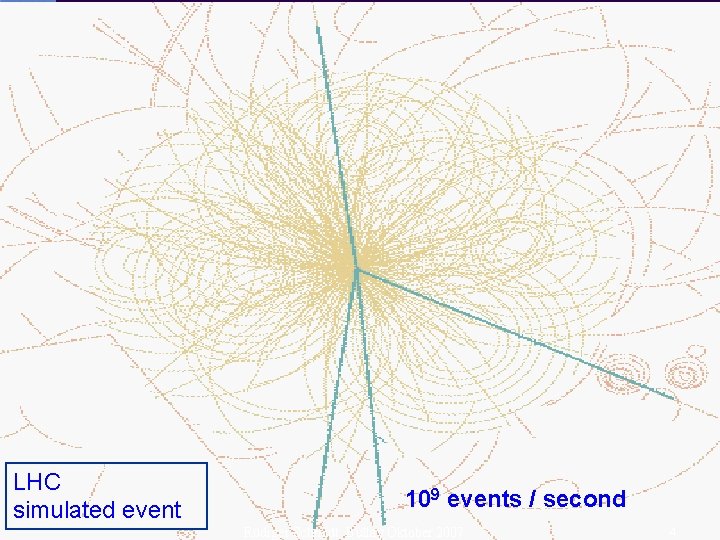
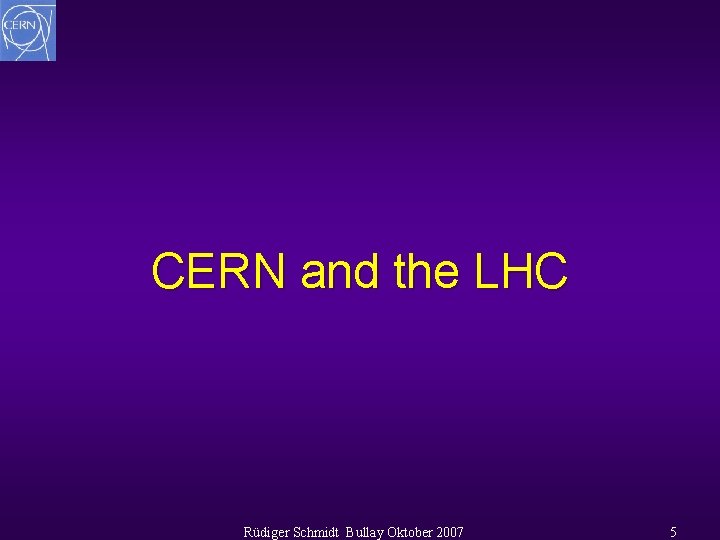
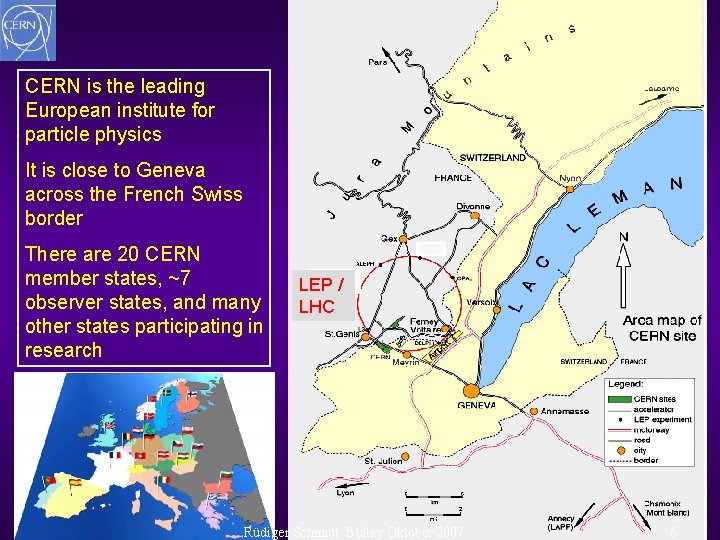
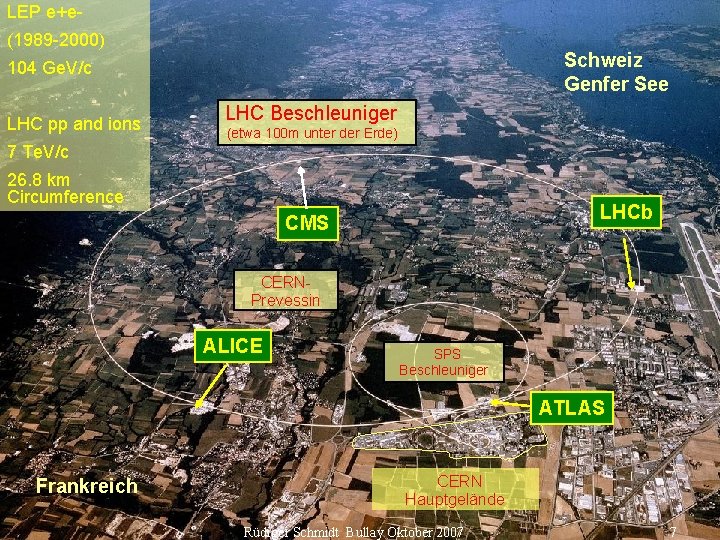
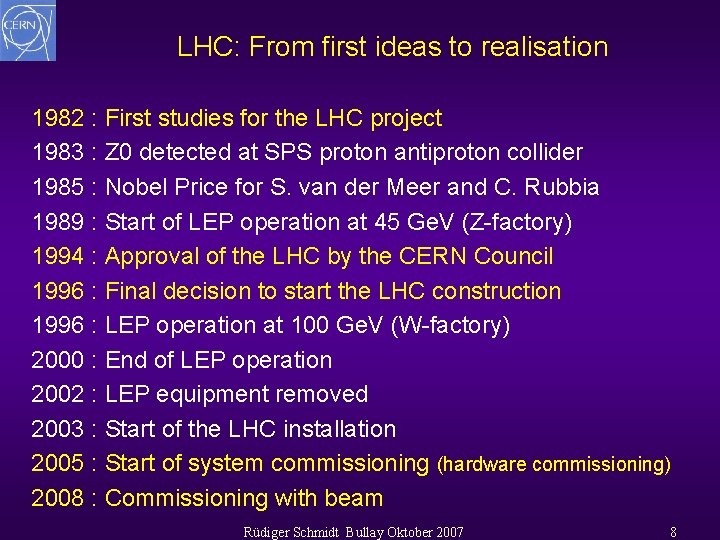
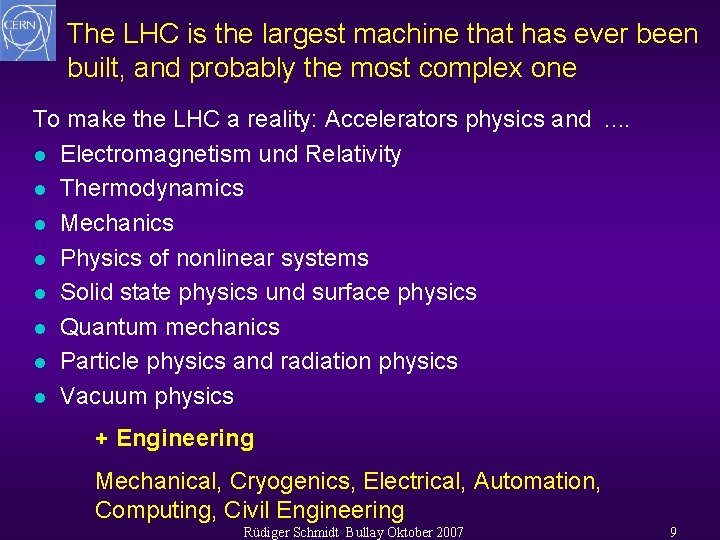
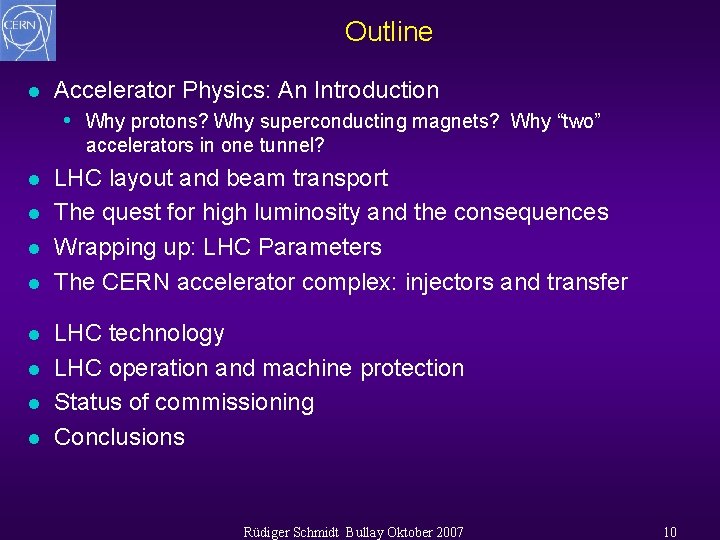
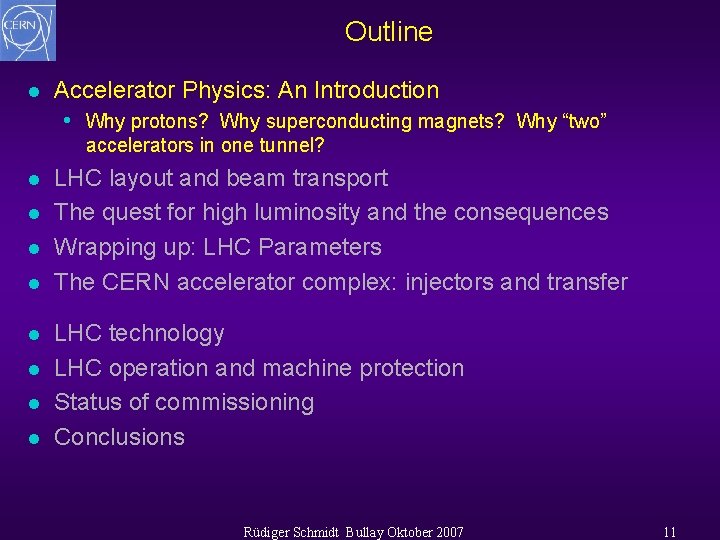
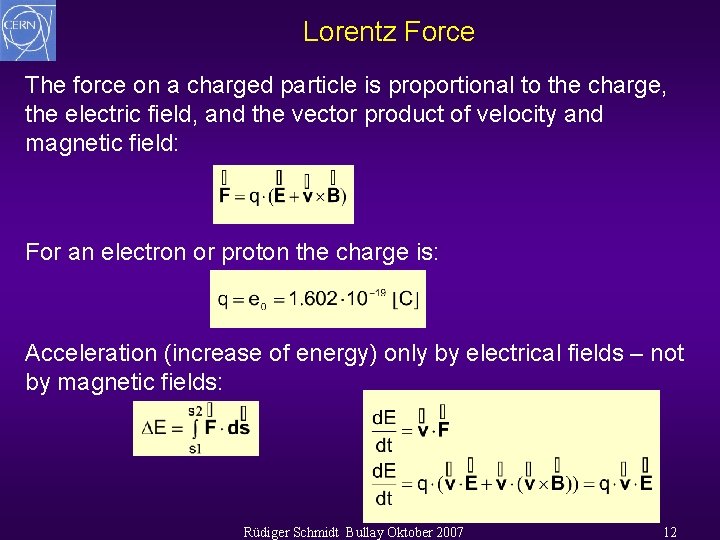
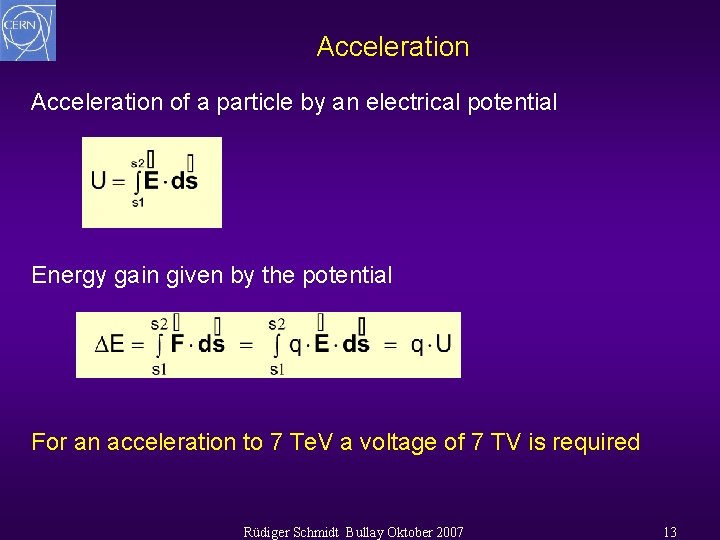
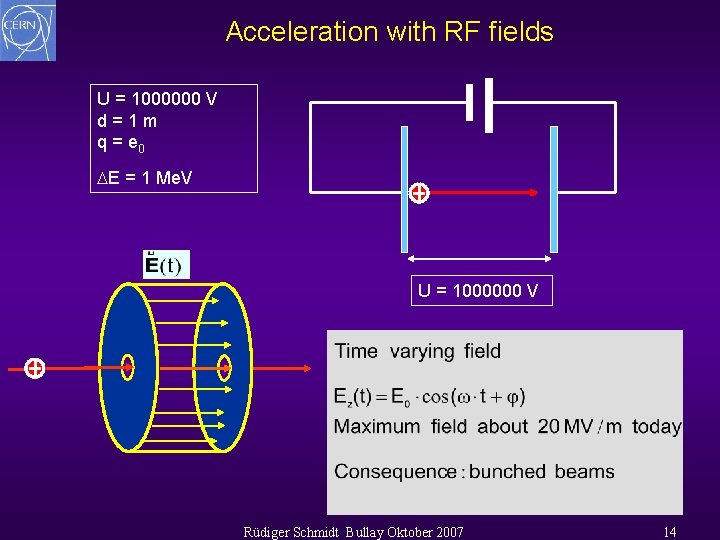
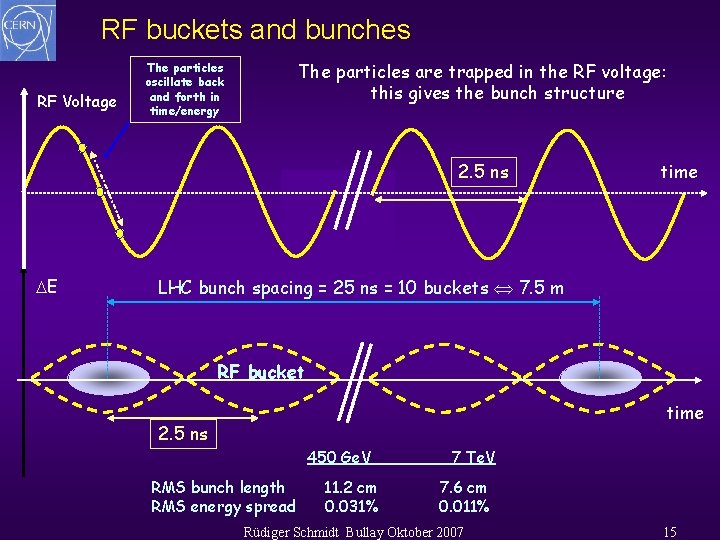
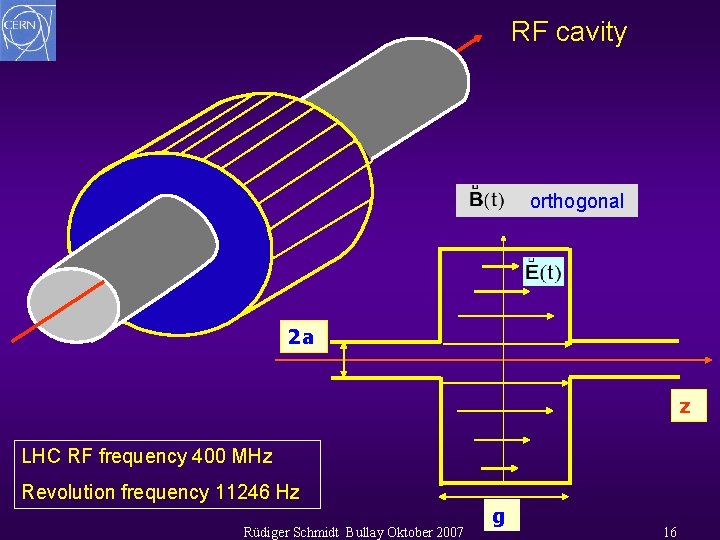
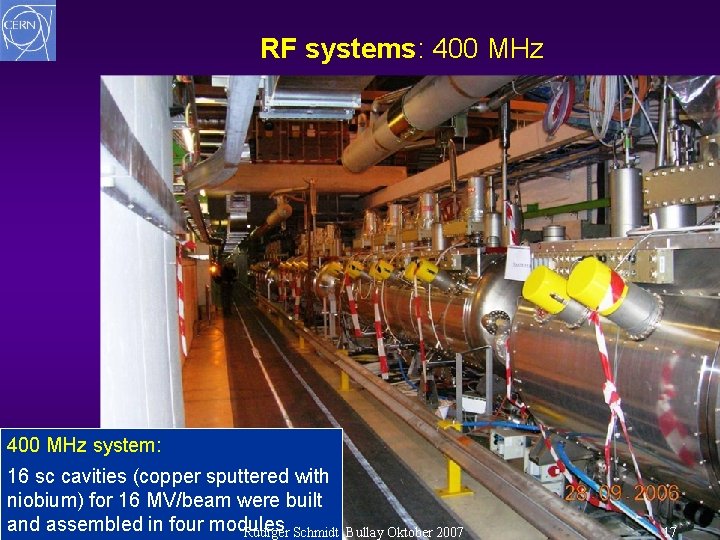
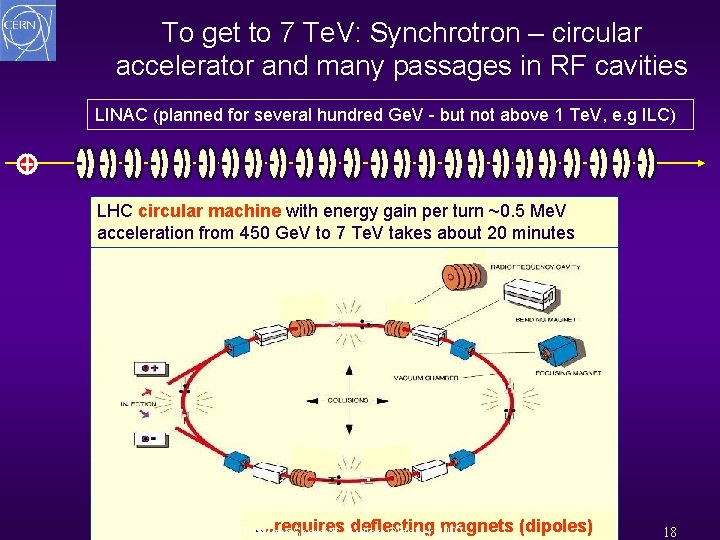
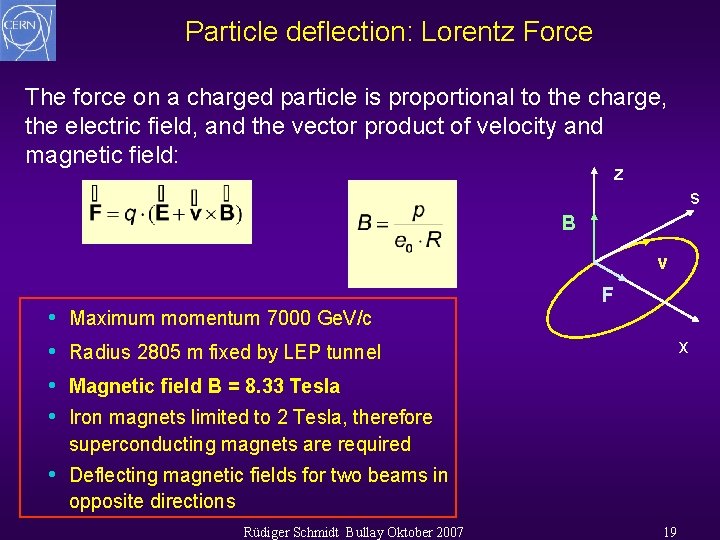
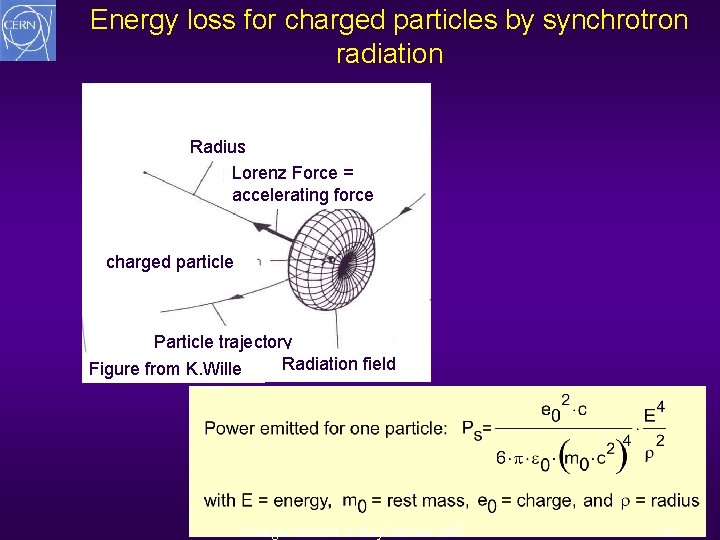
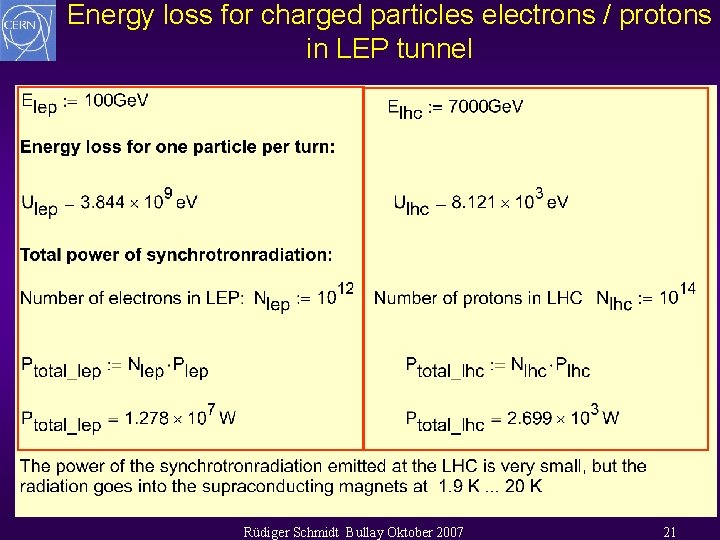
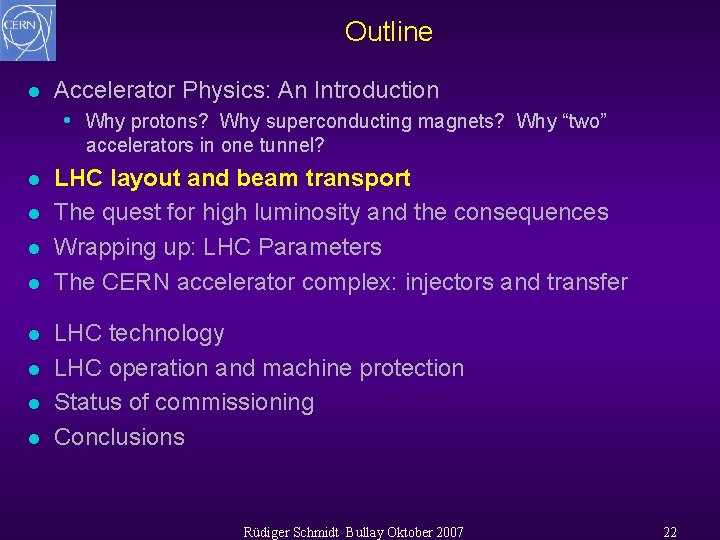
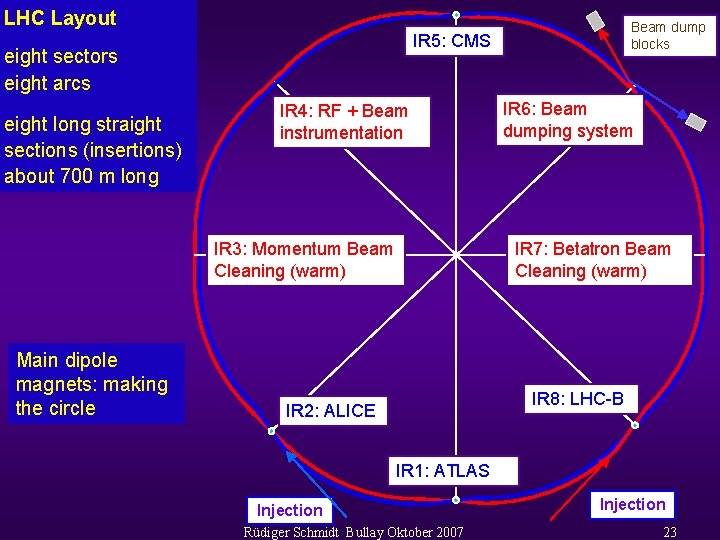
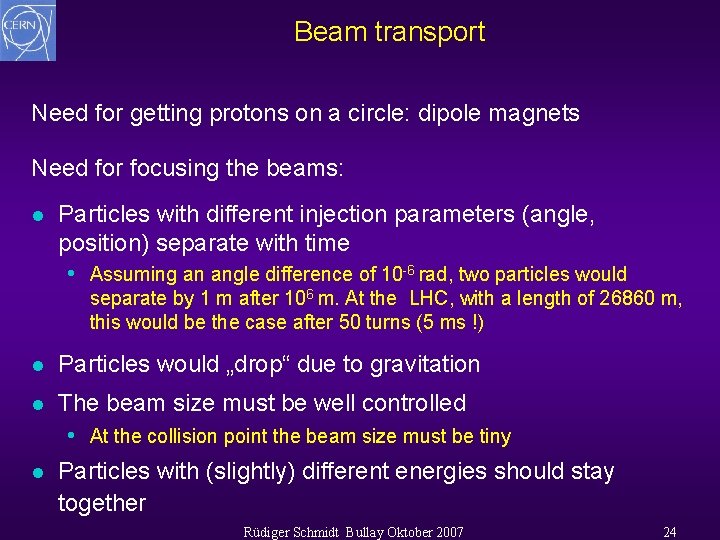
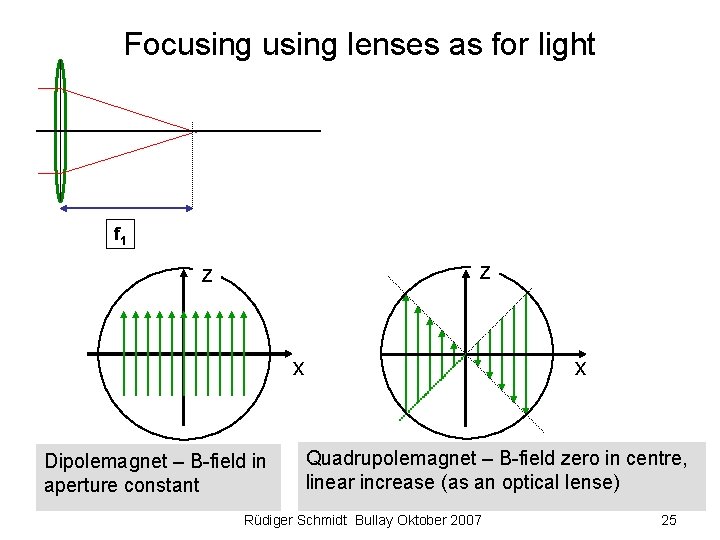
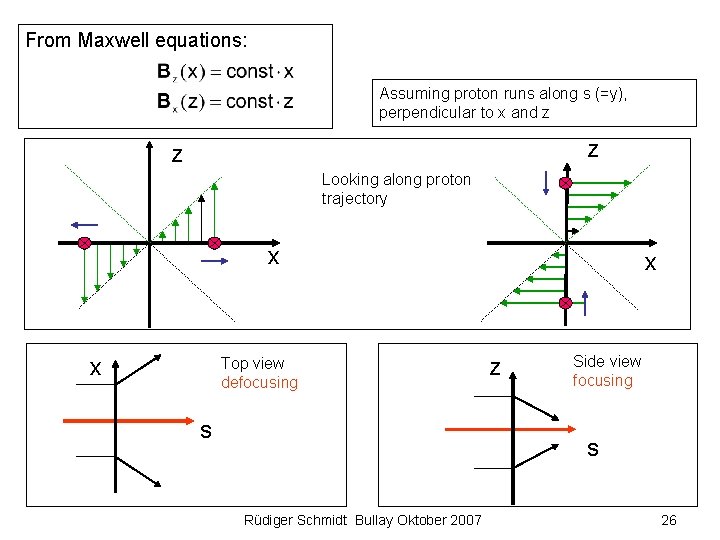
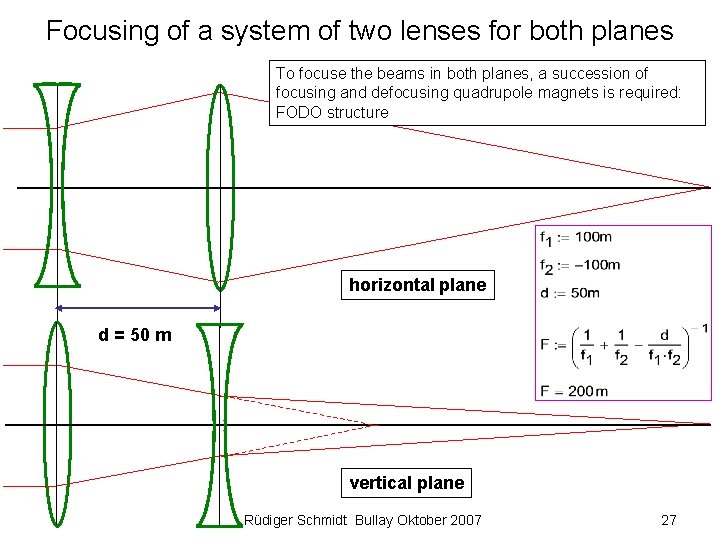
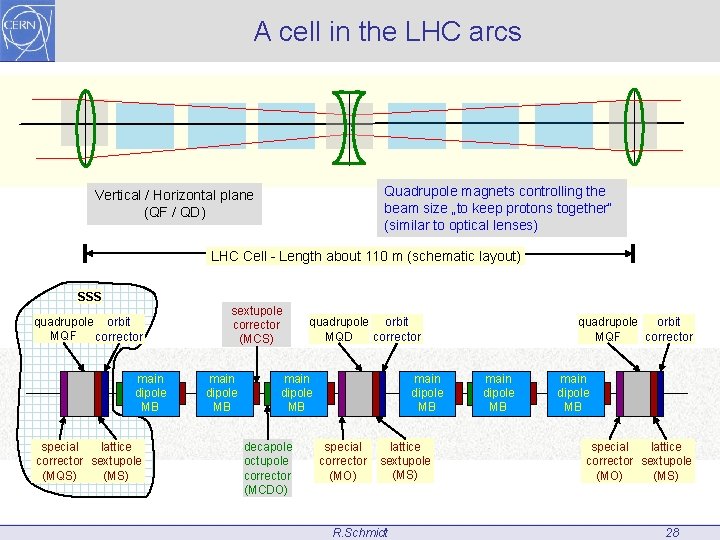
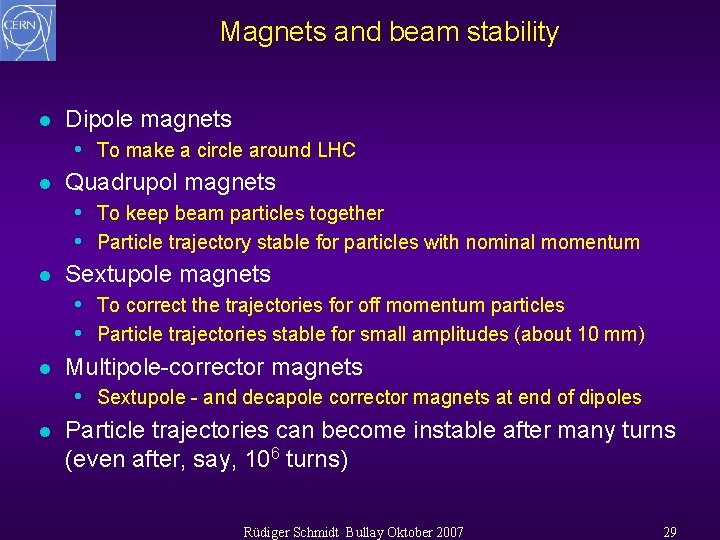
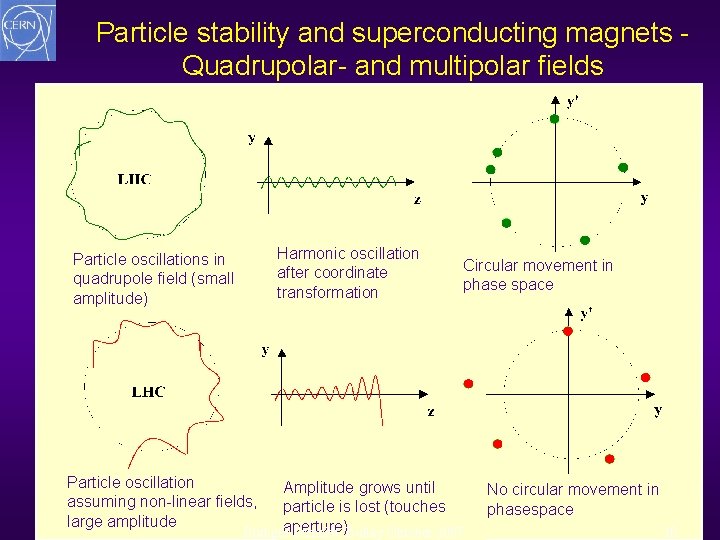
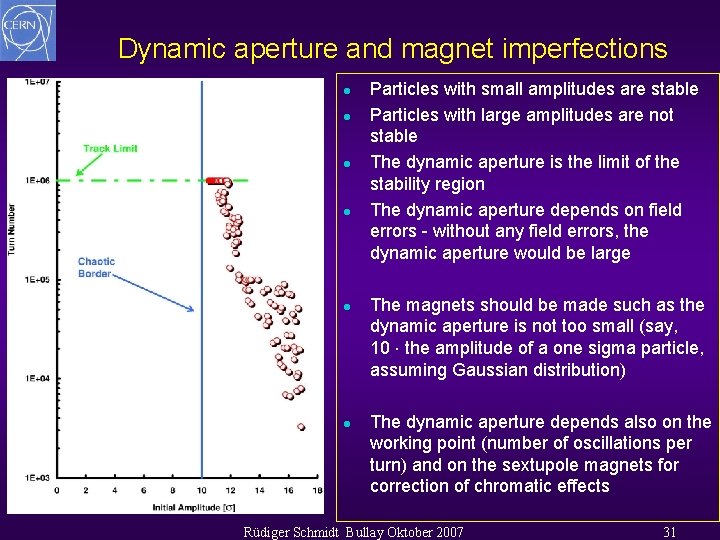
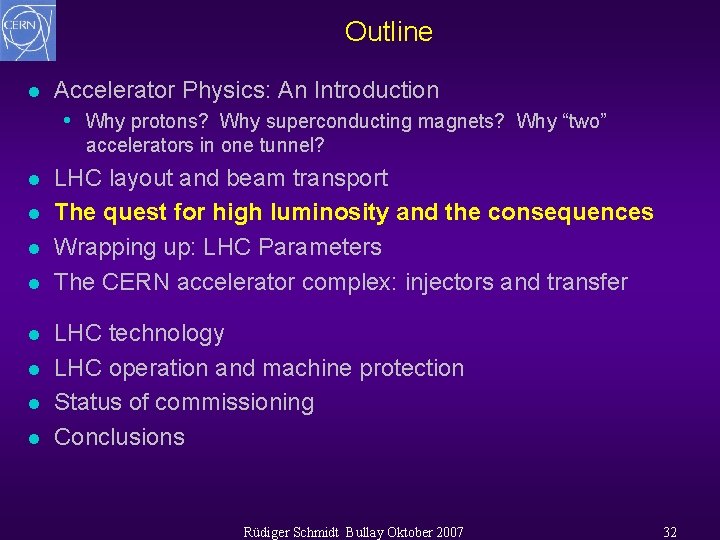
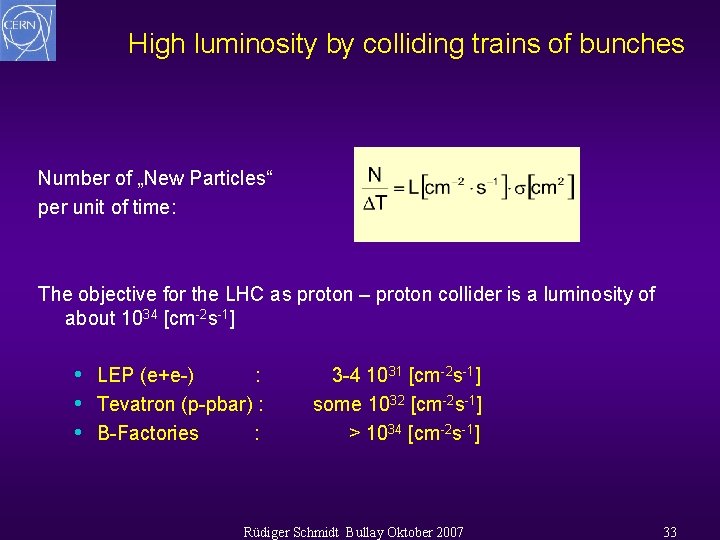
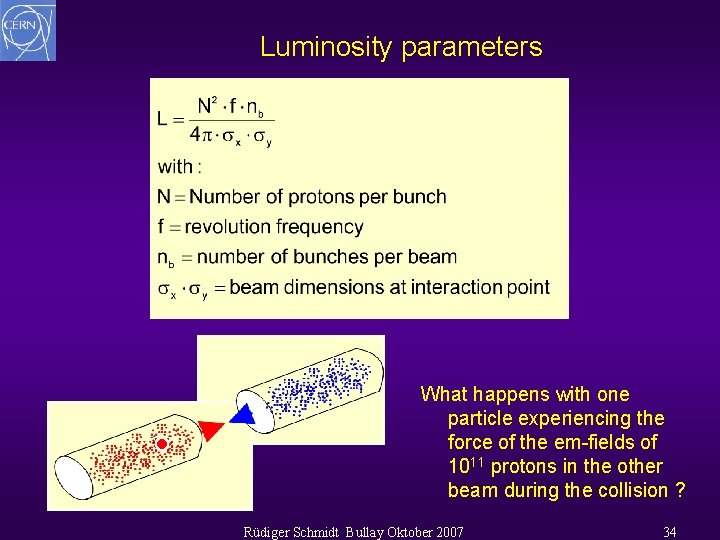
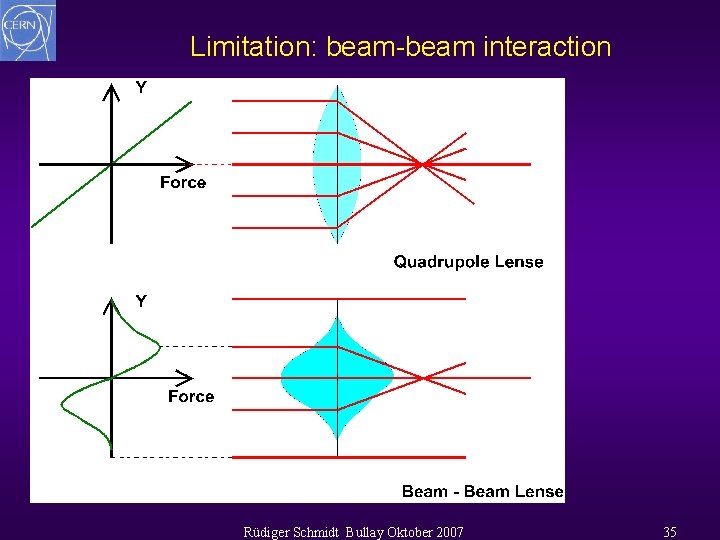
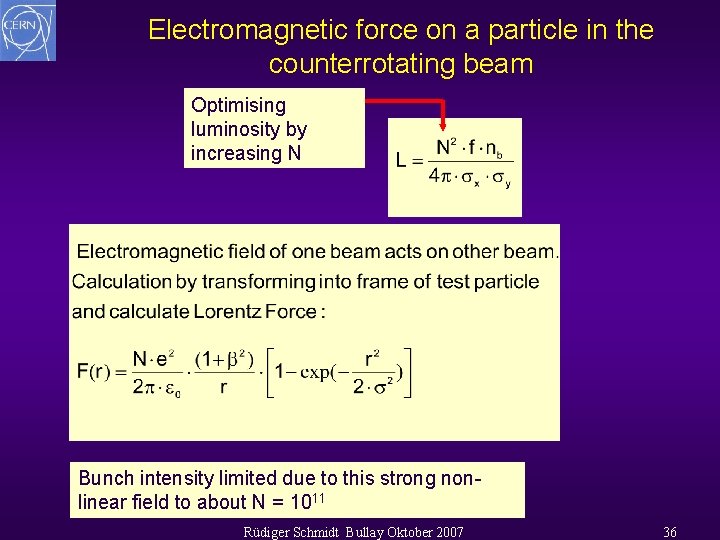
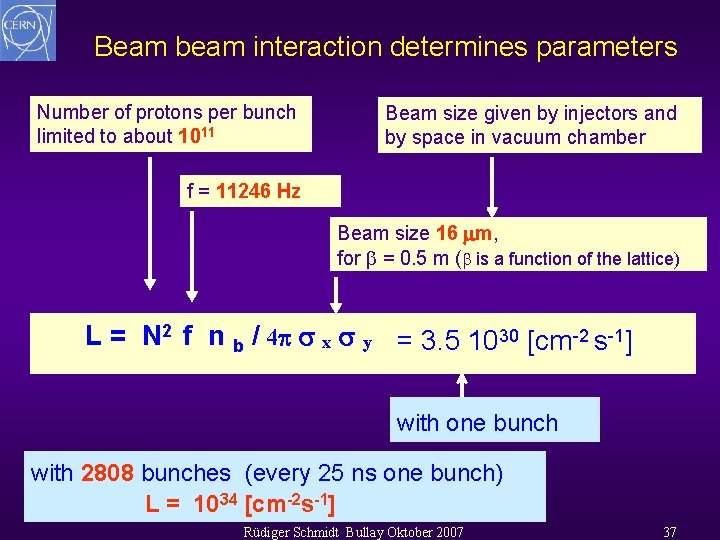
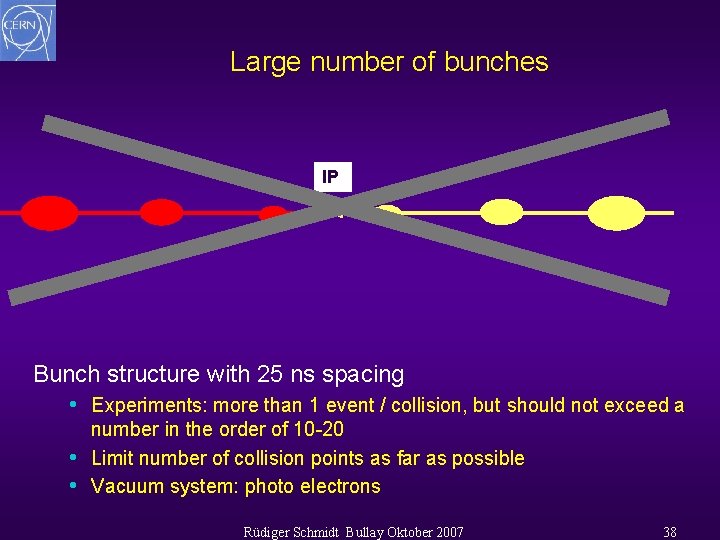
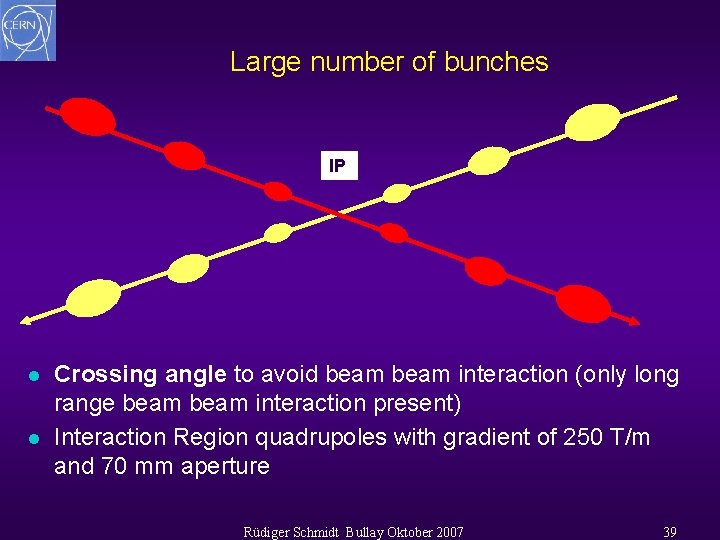
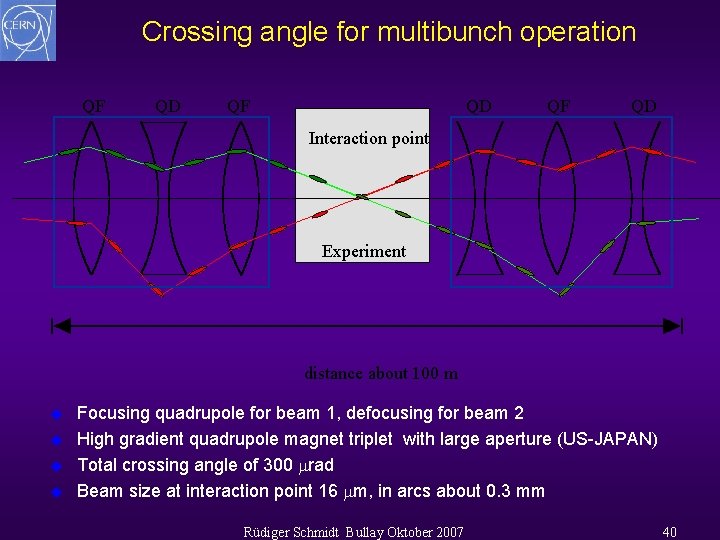
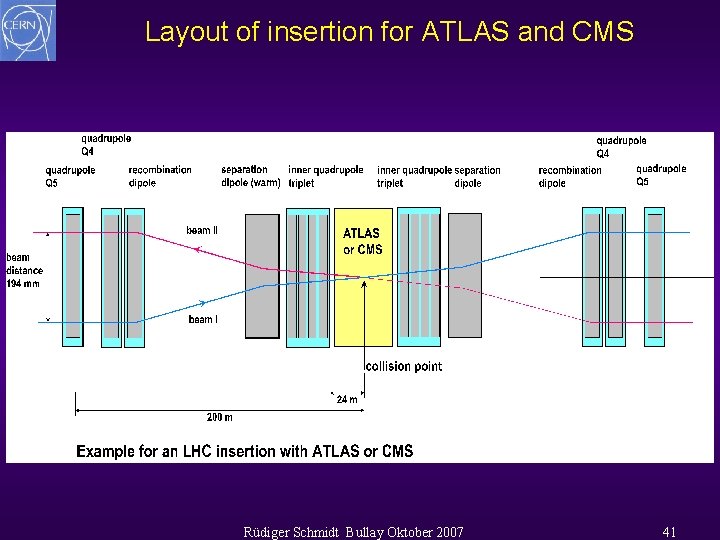
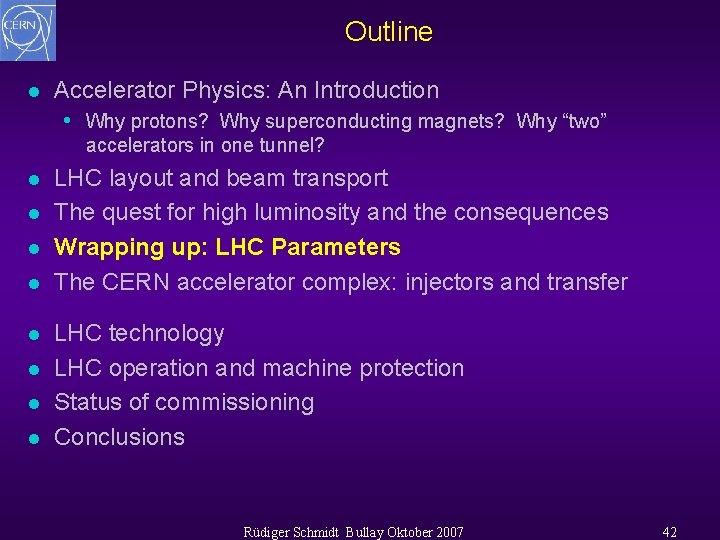
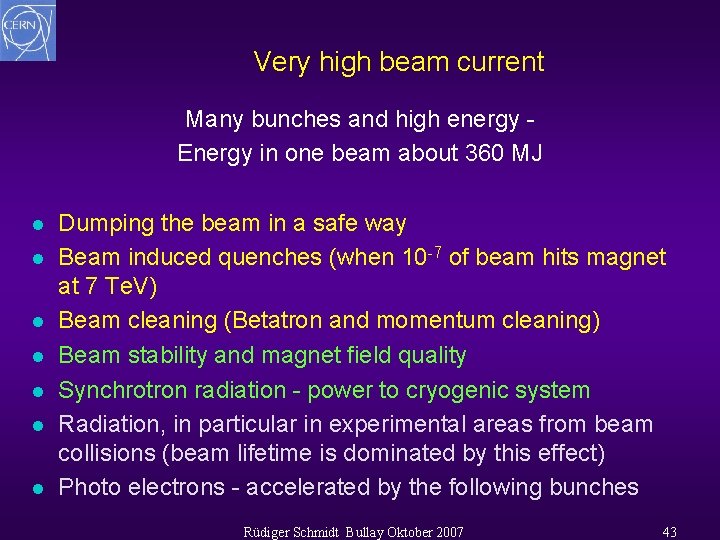
![Challenges: Energy stored in the beam [MJ] One beam, nominal intensity (corresponds to an Challenges: Energy stored in the beam [MJ] One beam, nominal intensity (corresponds to an](https://slidetodoc.com/presentation_image_h2/f8af96389038b62d47681788c18d4c7e/image-44.jpg)
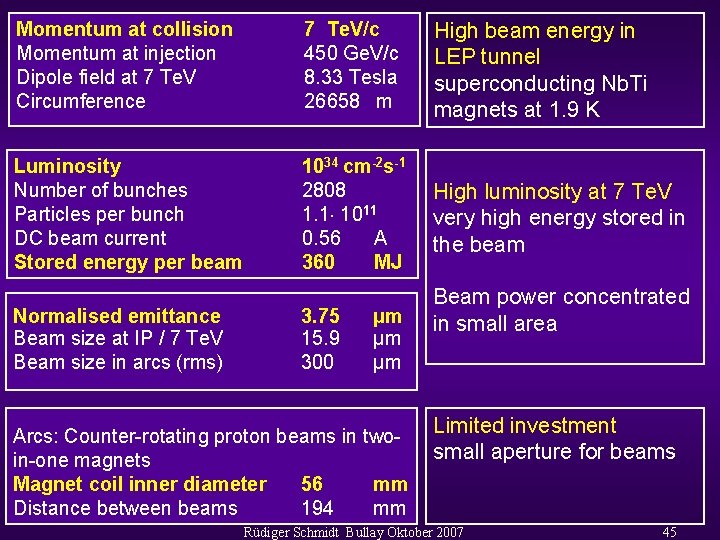
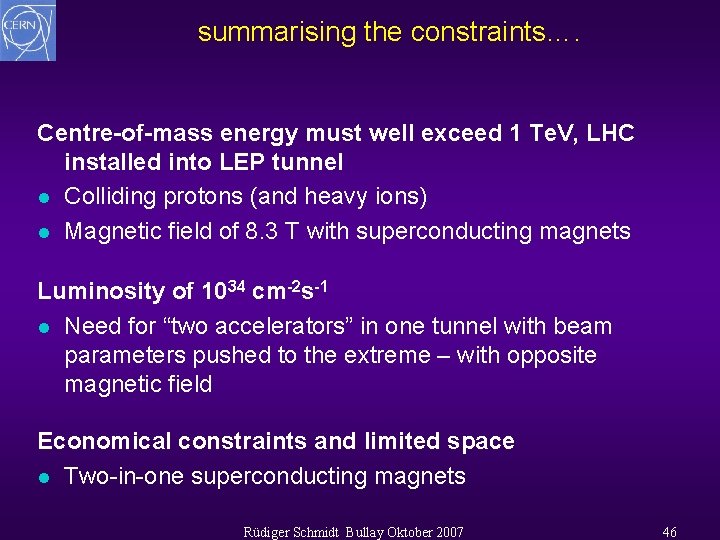
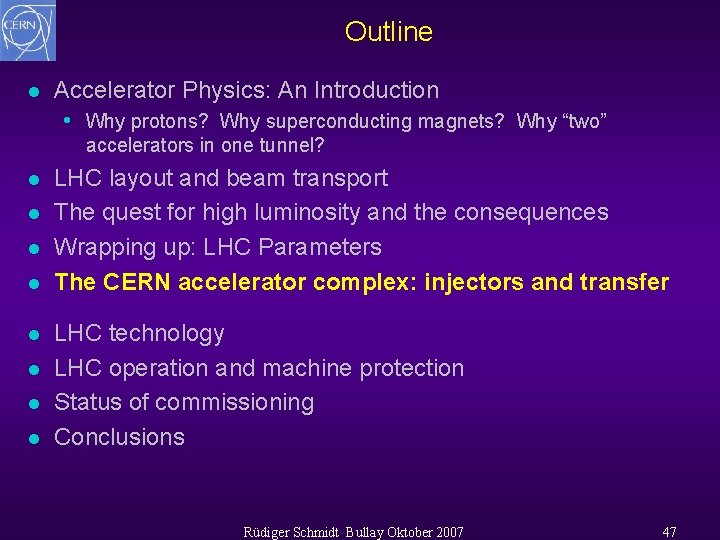
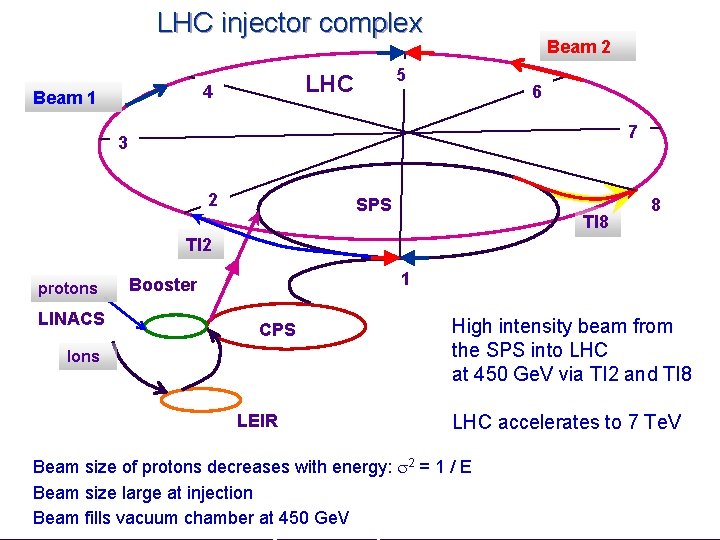
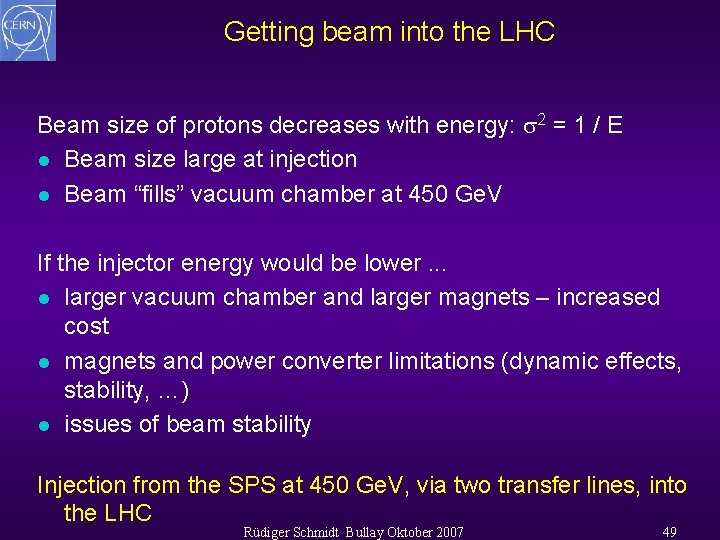
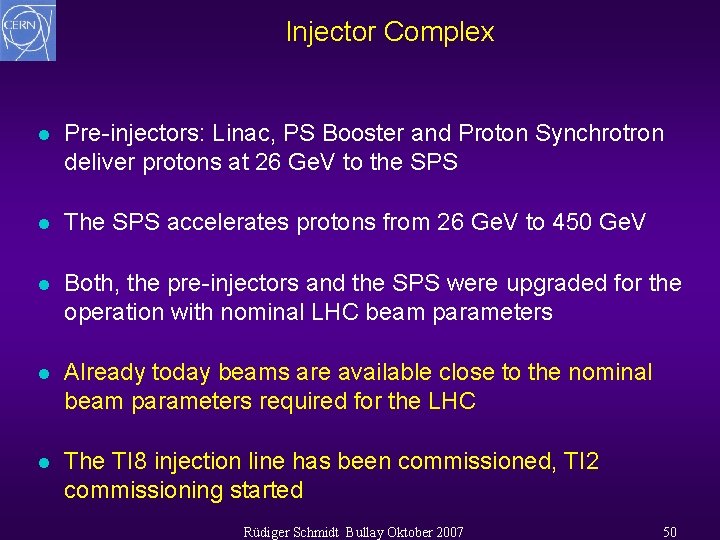
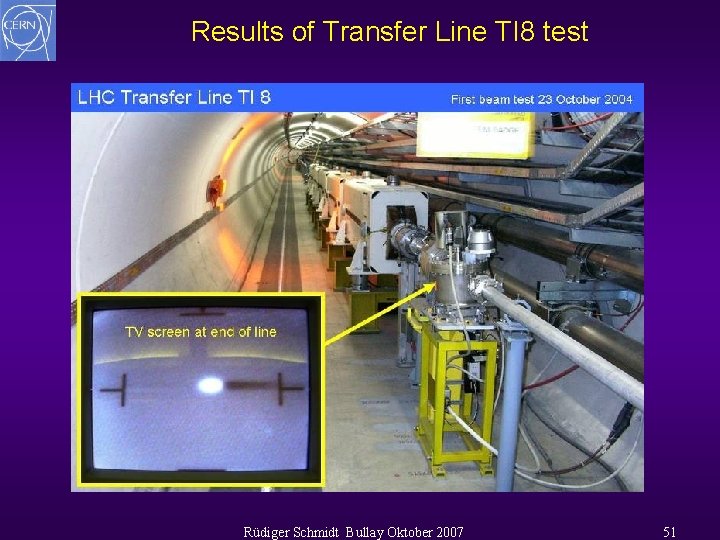
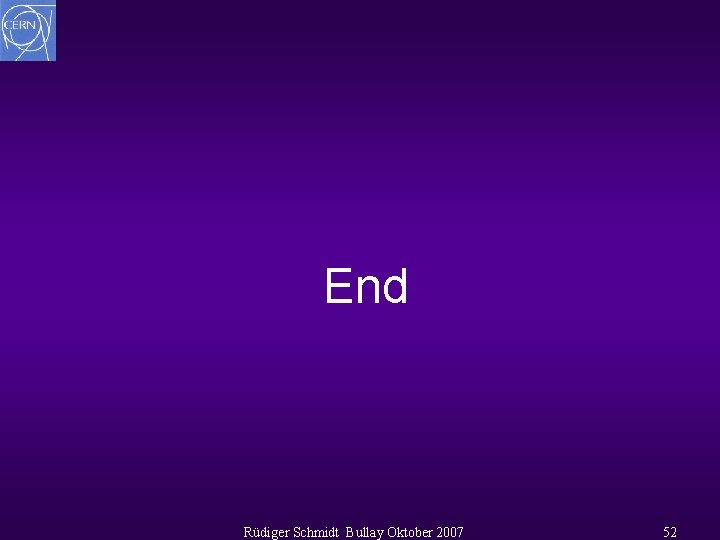
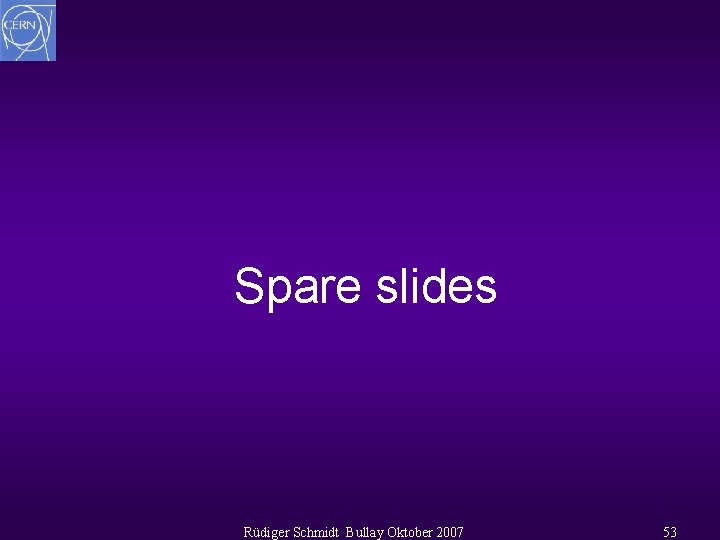
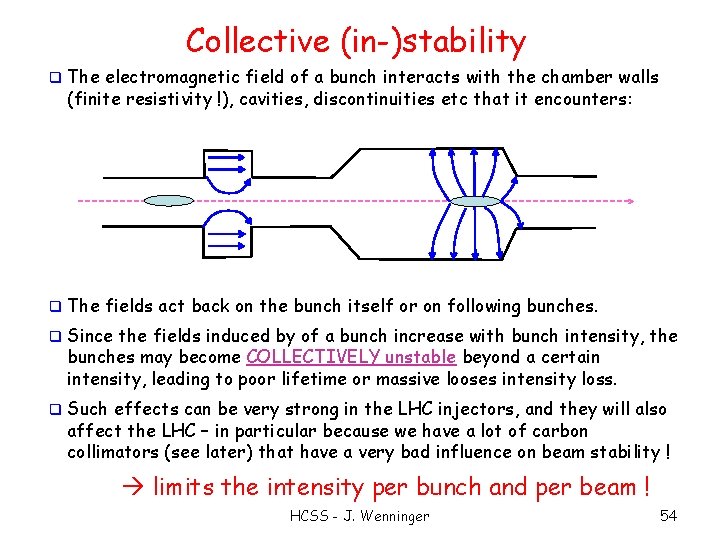
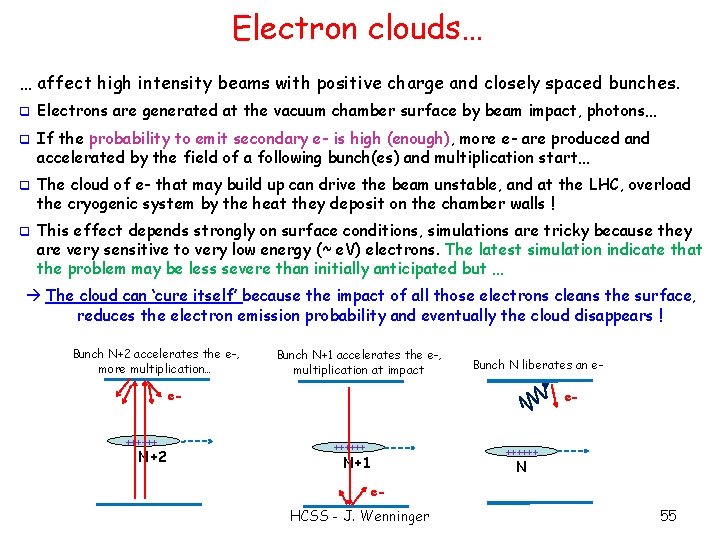
- Slides: 55
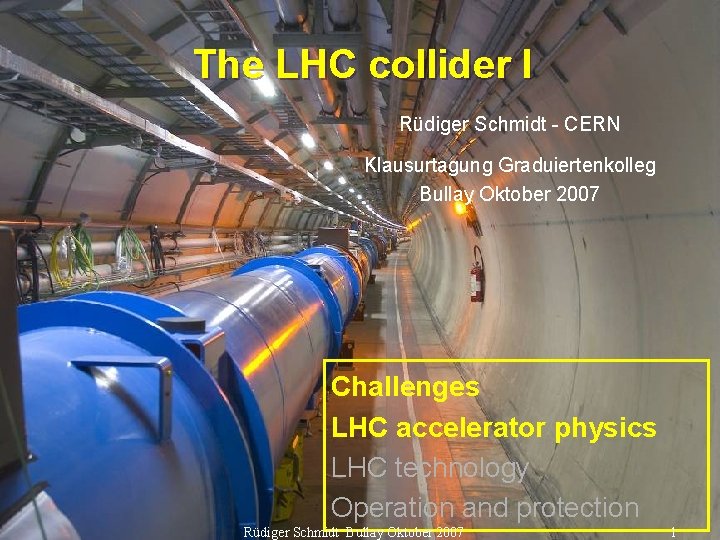
The LHC collider I Rüdiger Schmidt - CERN Klausurtagung Graduiertenkolleg Bullay Oktober 2007 Challenges LHC accelerator physics LHC technology Operation and protection Rüdiger Schmidt Bullay Oktober 2007 1
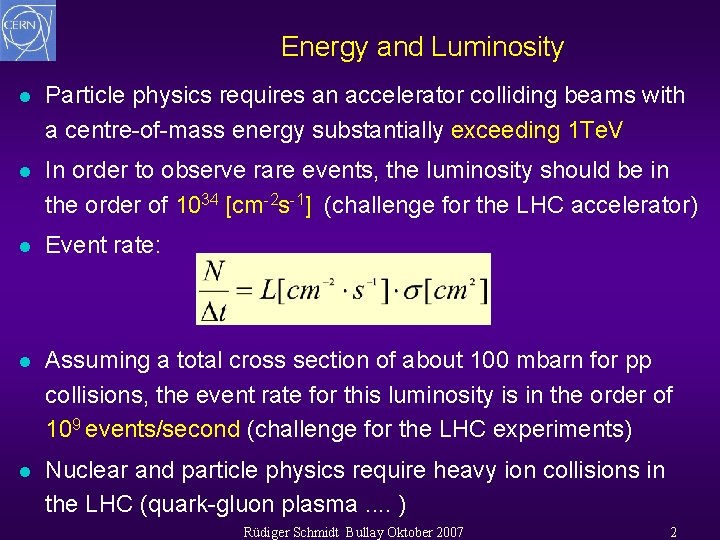
Energy and Luminosity l Particle physics requires an accelerator colliding beams with a centre-of-mass energy substantially exceeding 1 Te. V l In order to observe rare events, the luminosity should be in the order of 1034 [cm-2 s-1] (challenge for the LHC accelerator) l Event rate: l Assuming a total cross section of about 100 mbarn for pp collisions, the event rate for this luminosity is in the order of 109 events/second (challenge for the LHC experiments) l Nuclear and particle physics require heavy ion collisions in the LHC (quark-gluon plasma. . ) Rüdiger Schmidt Bullay Oktober 2007 2
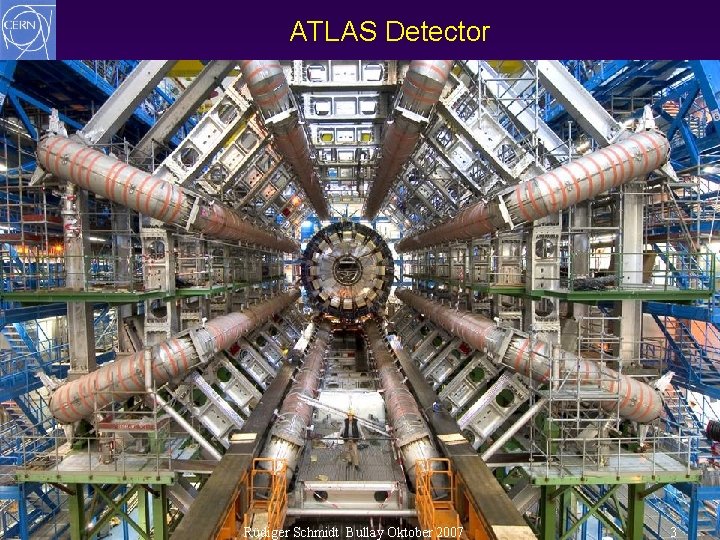
ATLAS Detector Rüdiger Schmidt Bullay Oktober 2007 3
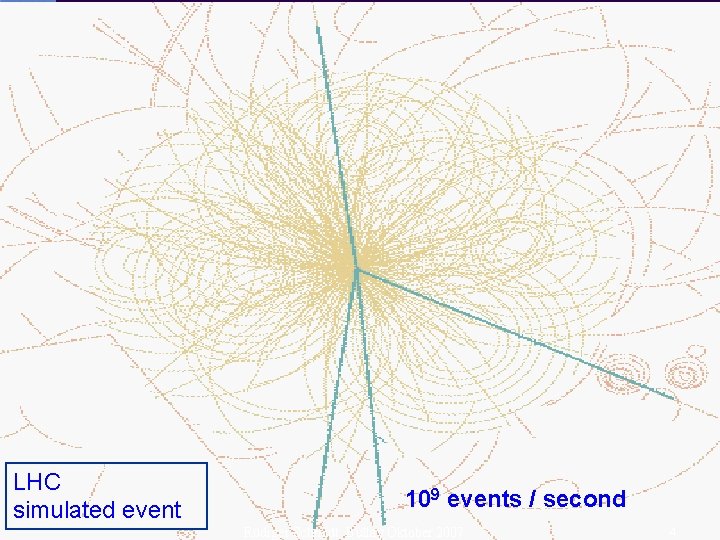
LHC simulated event 109 events / second Rüdiger Schmidt Bullay Oktober 2007 4
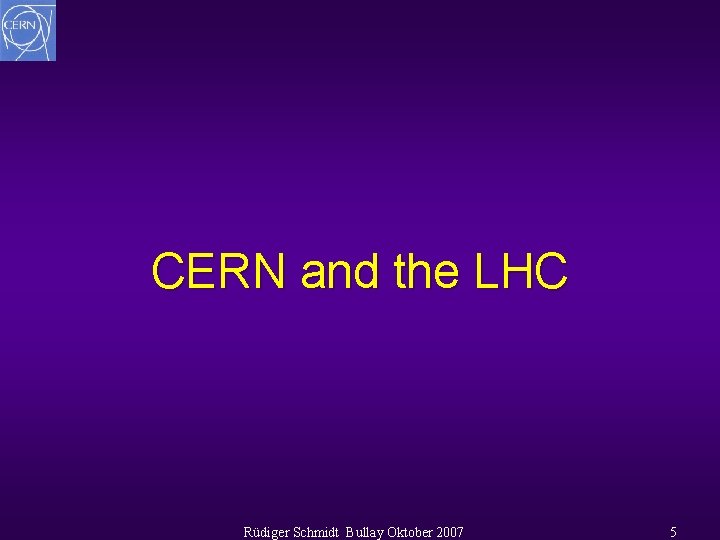
CERN and the LHC Rüdiger Schmidt Bullay Oktober 2007 5
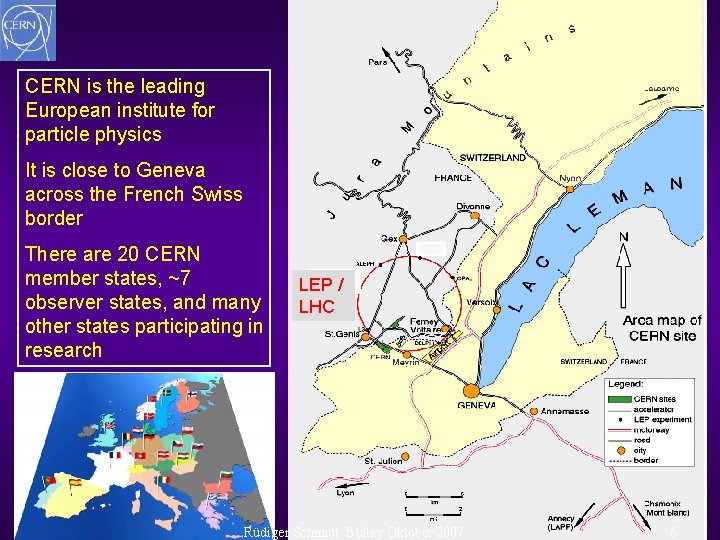
CERN is the leading European institute for particle physics It is close to Geneva across the French Swiss border There are 20 CERN member states, ~7 observer states, and many other states participating in research LEP / LHC Rüdiger Schmidt Bullay Oktober 2007 6
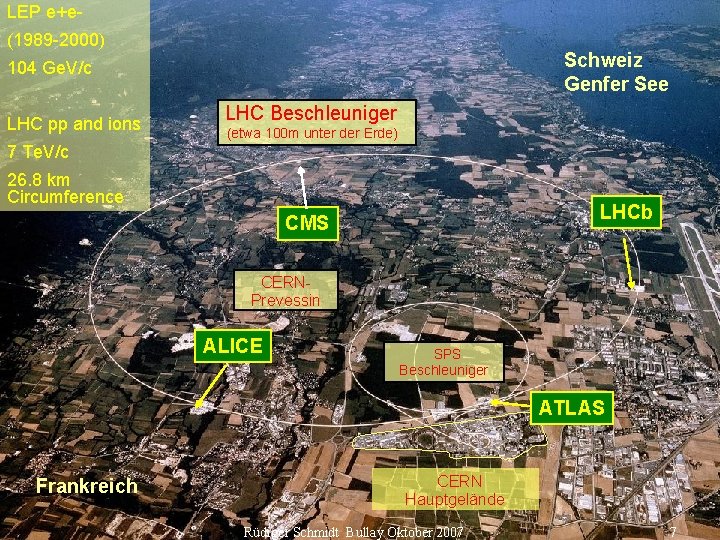
LEP e+e(1989 -2000) The CERN Beschleuniger Komplex Schweiz Genfer See 104 Ge. V/c LHC pp and ions LHC Beschleuniger (etwa 100 m unter der Erde) 7 Te. V/c 26. 8 km Circumference LHCb CMS CERNPrevessin ALICE SPS Beschleuniger ATLAS Frankreich CERN Hauptgelände Rüdiger Schmidt Bullay Oktober 2007 7
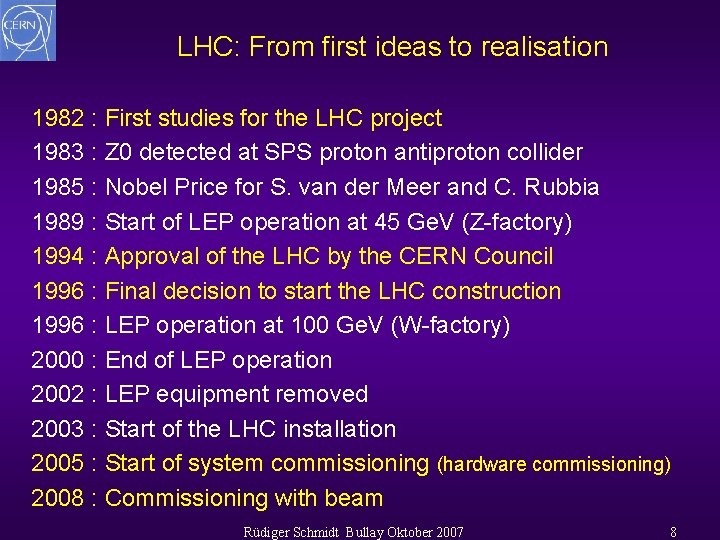
LHC: From first ideas to realisation 1982 : First studies for the LHC project 1983 : Z 0 detected at SPS proton antiproton collider 1985 : Nobel Price for S. van der Meer and C. Rubbia 1989 : Start of LEP operation at 45 Ge. V (Z-factory) 1994 : Approval of the LHC by the CERN Council 1996 : Final decision to start the LHC construction 1996 : LEP operation at 100 Ge. V (W-factory) 2000 : End of LEP operation 2002 : LEP equipment removed 2003 : Start of the LHC installation 2005 : Start of system commissioning (hardware commissioning) 2008 : Commissioning with beam Rüdiger Schmidt Bullay Oktober 2007 8
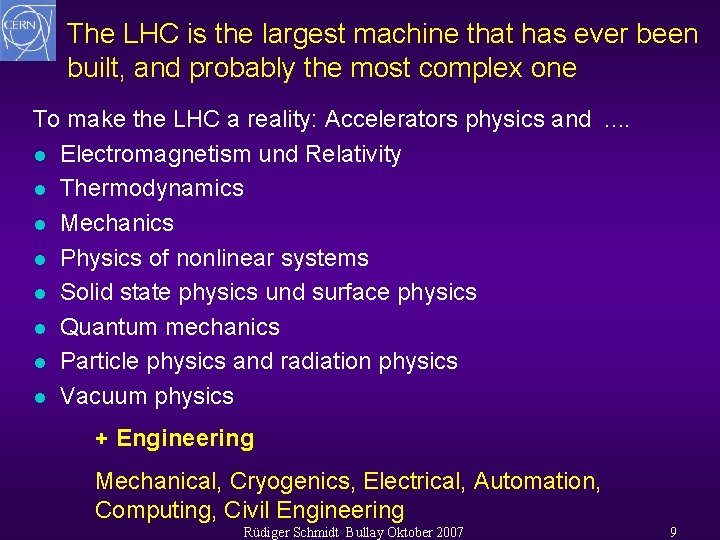
The LHC is the largest machine that has ever been built, and probably the most complex one To make the LHC a reality: Accelerators physics and. . l Electromagnetism und Relativity l Thermodynamics l Mechanics l Physics of nonlinear systems l Solid state physics und surface physics l Quantum mechanics l Particle physics and radiation physics l Vacuum physics + Engineering Mechanical, Cryogenics, Electrical, Automation, Computing, Civil Engineering Rüdiger Schmidt Bullay Oktober 2007 9
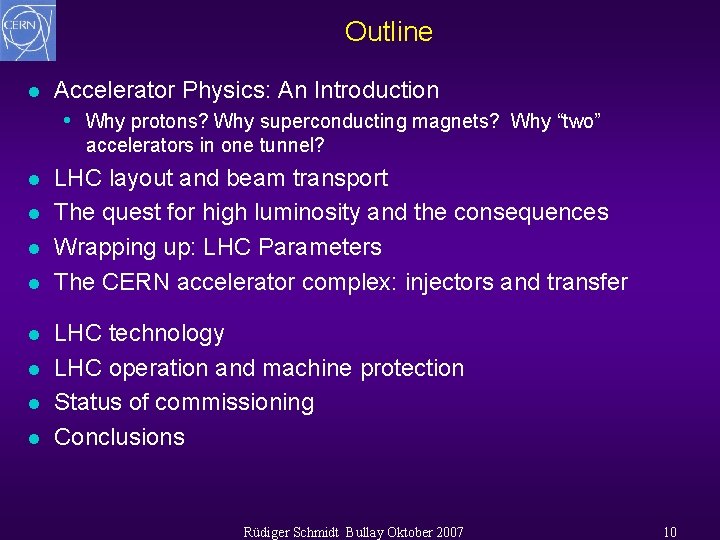
Outline l Accelerator Physics: An Introduction • Why protons? Why superconducting magnets? Why “two” accelerators in one tunnel? l l l l LHC layout and beam transport The quest for high luminosity and the consequences Wrapping up: LHC Parameters The CERN accelerator complex: injectors and transfer LHC technology LHC operation and machine protection Status of commissioning Conclusions Rüdiger Schmidt Bullay Oktober 2007 10
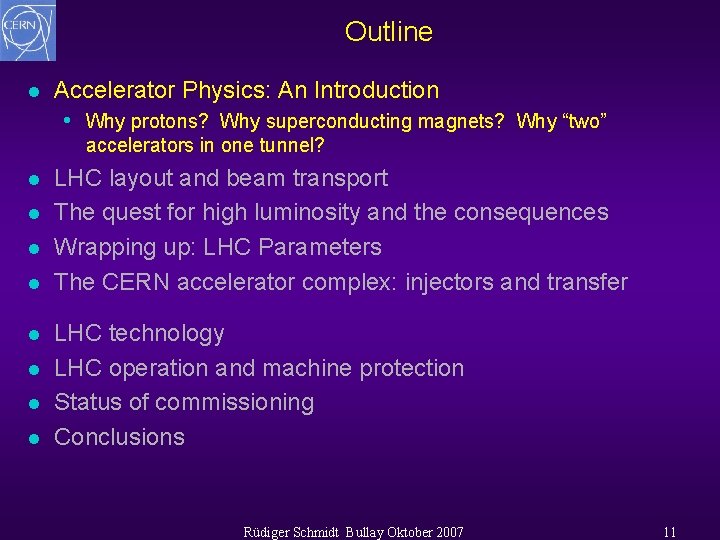
Outline l Accelerator Physics: An Introduction • Why protons? Why superconducting magnets? Why “two” accelerators in one tunnel? l l l l LHC layout and beam transport The quest for high luminosity and the consequences Wrapping up: LHC Parameters The CERN accelerator complex: injectors and transfer LHC technology LHC operation and machine protection Status of commissioning Conclusions Rüdiger Schmidt Bullay Oktober 2007 11
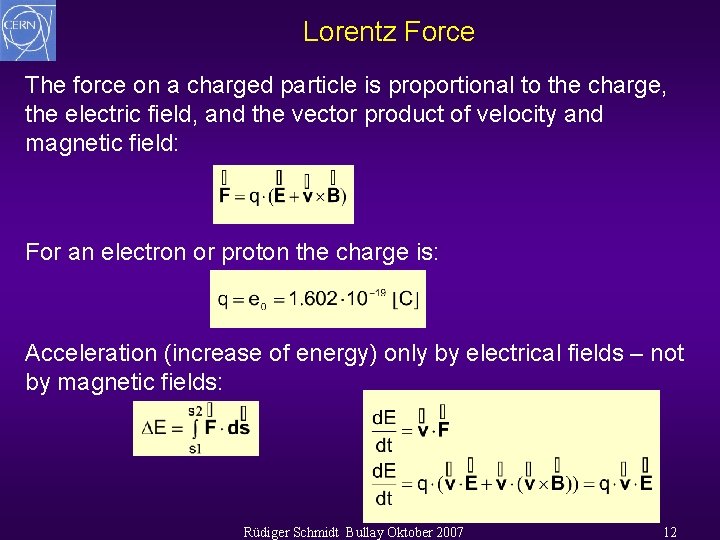
Lorentz Force The force on a charged particle is proportional to the charge, the electric field, and the vector product of velocity and magnetic field: For an electron or proton the charge is: Acceleration (increase of energy) only by electrical fields – not by magnetic fields: Rüdiger Schmidt Bullay Oktober 2007 12
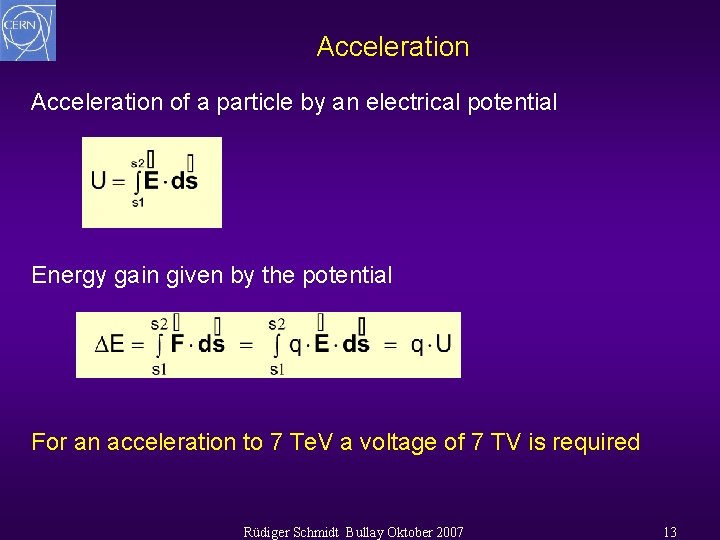
Acceleration of a particle by an electrical potential Energy gain given by the potential For an acceleration to 7 Te. V a voltage of 7 TV is required Rüdiger Schmidt Bullay Oktober 2007 13
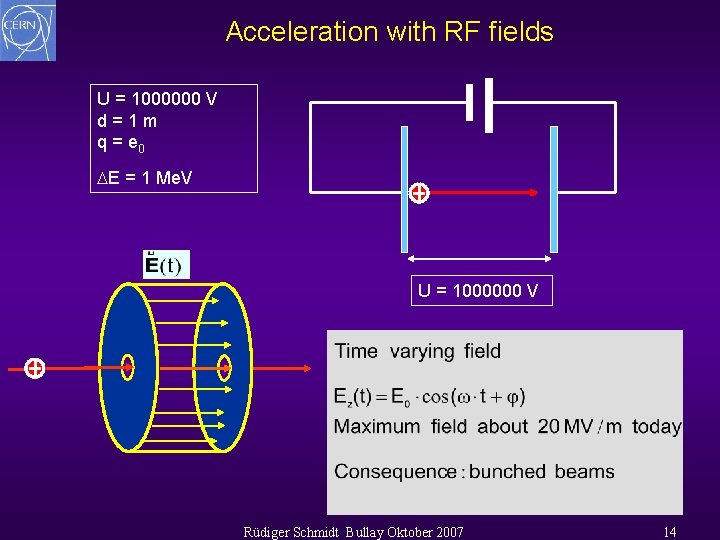
Acceleration with RF fields U = 1000000 V d=1 m q = e 0 E = 1 Me. V U = 1000000 V Rüdiger Schmidt Bullay Oktober 2007 14
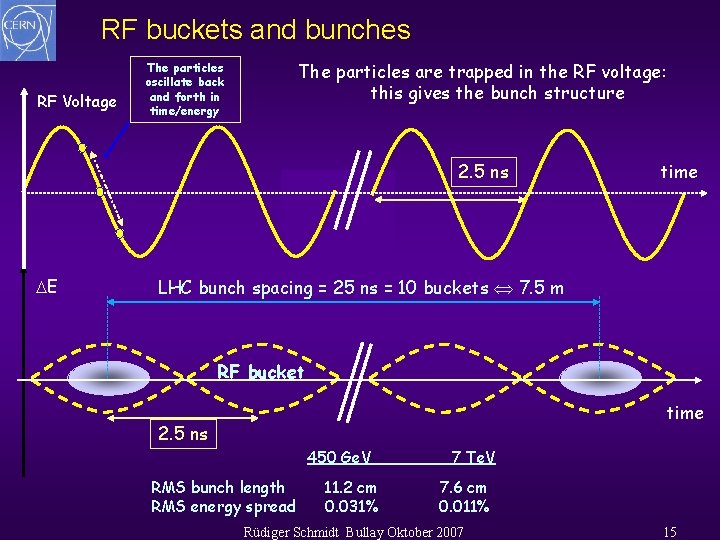
RF buckets and bunches RF Voltage The particles are trapped in the RF voltage: this gives the bunch structure The particles oscillate back and forth in time/energy 2. 5 ns E time LHC bunch spacing = 25 ns = 10 buckets 7. 5 m RF bucket time 2. 5 ns 450 Ge. V RMS bunch length RMS energy spread 11. 2 cm 0. 031% 7 Te. V 7. 6 cm 0. 011% Rüdiger Schmidt Bullay Oktober 2007 15
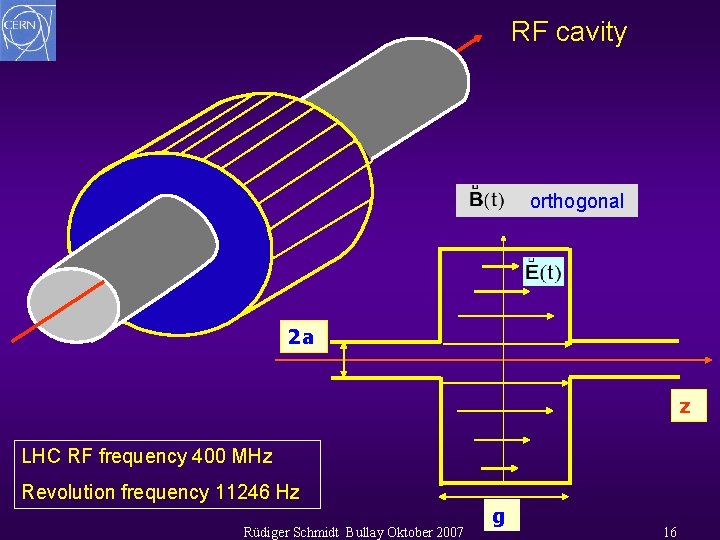
RF cavity orthogonal 2 a z LHC RF frequency 400 MHz Revolution frequency 11246 Hz Rüdiger Schmidt Bullay Oktober 2007 g 16
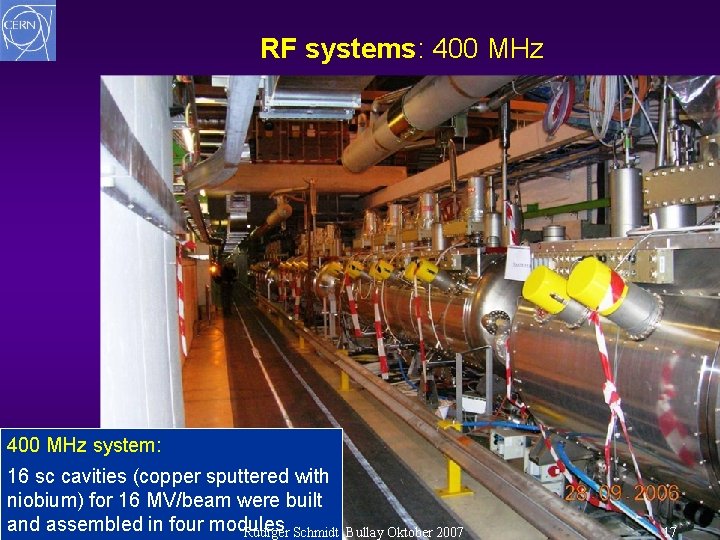
RF systems: 400 MHz system: 16 sc cavities (copper sputtered with niobium) for 16 MV/beam were built and assembled in four modules Rüdiger Schmidt Bullay Oktober 2007 17
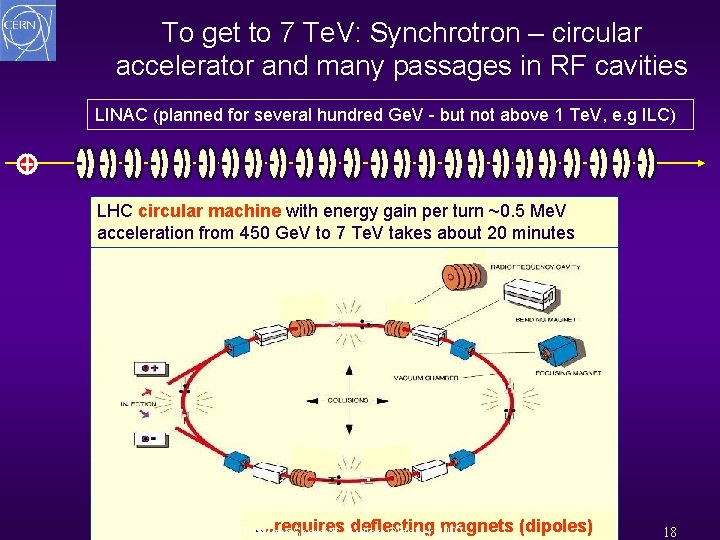
To get to 7 Te. V: Synchrotron – circular accelerator and many passages in RF cavities LINAC (planned for several hundred Ge. V - but not above 1 Te. V, e. g ILC) LHC circular machine with energy gain per turn ~0. 5 Me. V acceleration from 450 Ge. V to 7 Te. V takes about 20 minutes . . requires deflecting magnets Rüdiger Schmidt Bullay Oktober 2007 (dipoles) 18
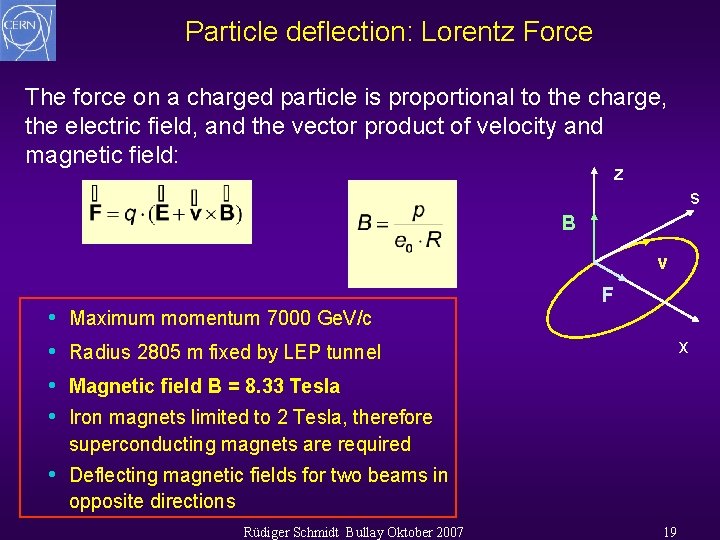
Particle deflection: Lorentz Force The force on a charged particle is proportional to the charge, the electric field, and the vector product of velocity and magnetic field: z s B v • • F Maximum momentum 7000 Ge. V/c x Radius 2805 m fixed by LEP tunnel Magnetic field B = 8. 33 Tesla Iron magnets limited to 2 Tesla, therefore superconducting magnets are required • Deflecting magnetic fields for two beams in opposite directions Rüdiger Schmidt Bullay Oktober 2007 19
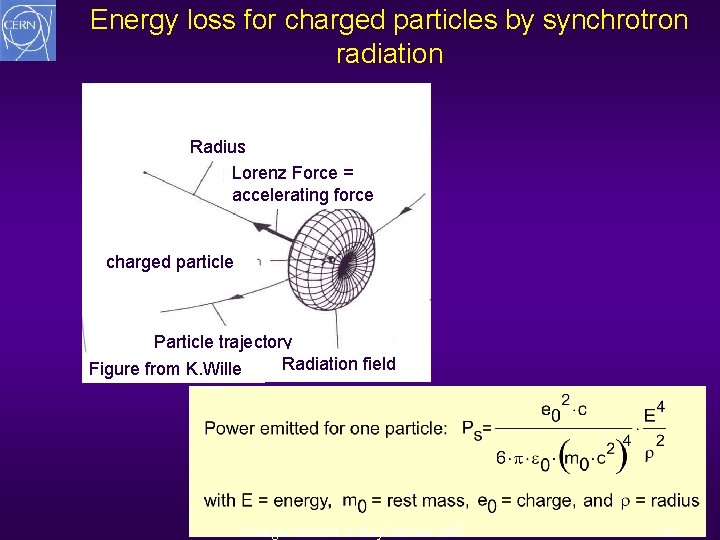
Energy loss for charged particles by synchrotron radiation Radius Lorenz Force = accelerating force charged particle Particle trajectory Radiation field Figure from K. Wille Rüdiger Schmidt Bullay Oktober 2007 20
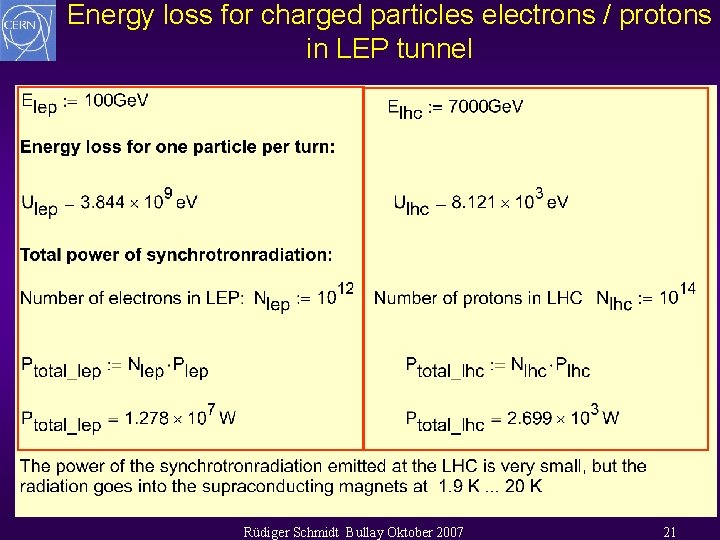
Energy loss for charged particles electrons / protons in LEP tunnel Rüdiger Schmidt Bullay Oktober 2007 21
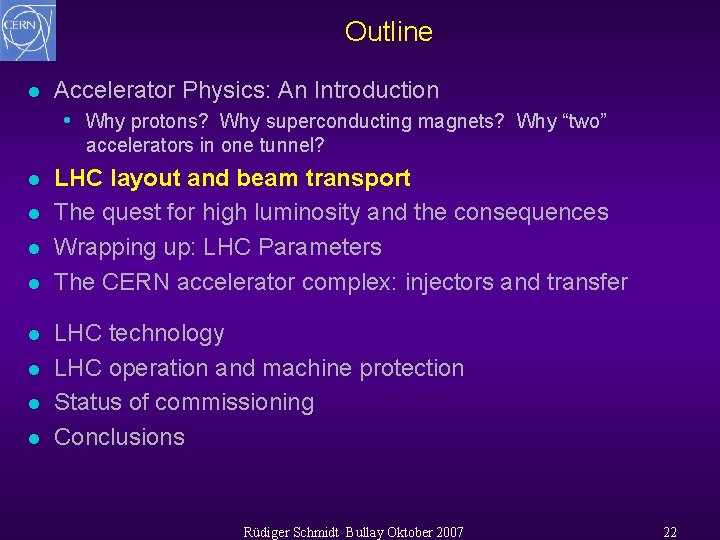
Outline l Accelerator Physics: An Introduction • Why protons? Why superconducting magnets? Why “two” accelerators in one tunnel? l l l l LHC layout and beam transport The quest for high luminosity and the consequences Wrapping up: LHC Parameters The CERN accelerator complex: injectors and transfer LHC technology LHC operation and machine protection Status of commissioning Conclusions Rüdiger Schmidt Bullay Oktober 2007 22
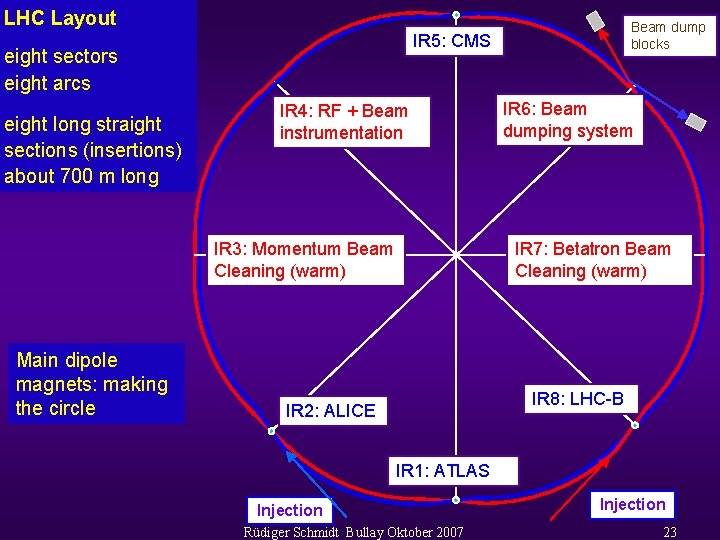
LHC Layout eight sectors eight arcs eight long straight sections (insertions) about 700 m long IR 4: RF + Beam instrumentation IR 3: Momentum Beam Cleaning (warm) Main dipole magnets: making the circle Beam dump blocks IR 5: CMS IR 6: Beam dumping system IR 7: Betatron Beam Cleaning (warm) IR 8: LHC-B IR 2: ALICE IR 1: ATLAS Injection Rüdiger Schmidt Bullay Oktober 2007 Injection 23
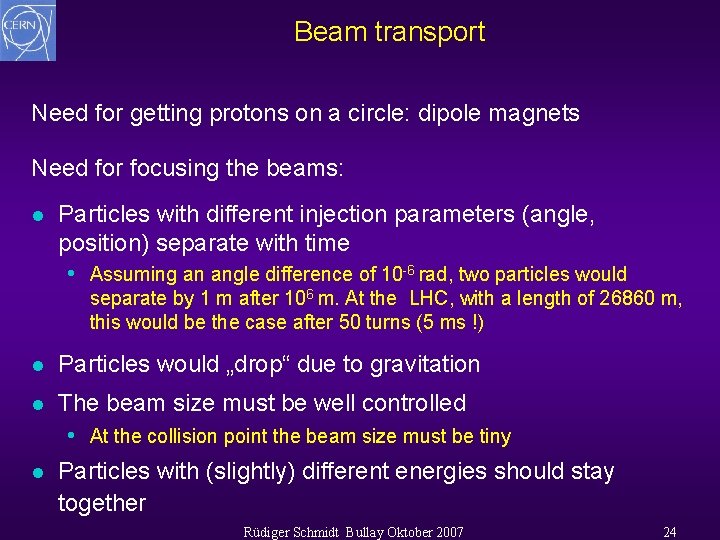
Beam transport Need for getting protons on a circle: dipole magnets Need for focusing the beams: l Particles with different injection parameters (angle, position) separate with time • Assuming an angle difference of 10 -6 rad, two particles would separate by 1 m after 106 m. At the LHC, with a length of 26860 m, this would be the case after 50 turns (5 ms !) l Particles would „drop“ due to gravitation l The beam size must be well controlled • At the collision point the beam size must be tiny l Particles with (slightly) different energies should stay together Rüdiger Schmidt Bullay Oktober 2007 24
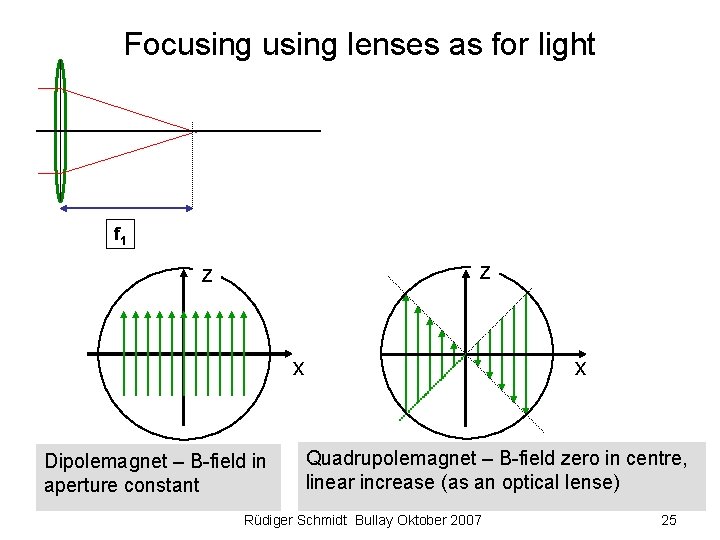
Focusing lenses as for light f 1 z z x Dipolemagnet – B-field in aperture constant x Quadrupolemagnet – B-field zero in centre, linear increase (as an optical lense) Rüdiger Schmidt Bullay Oktober 2007 25
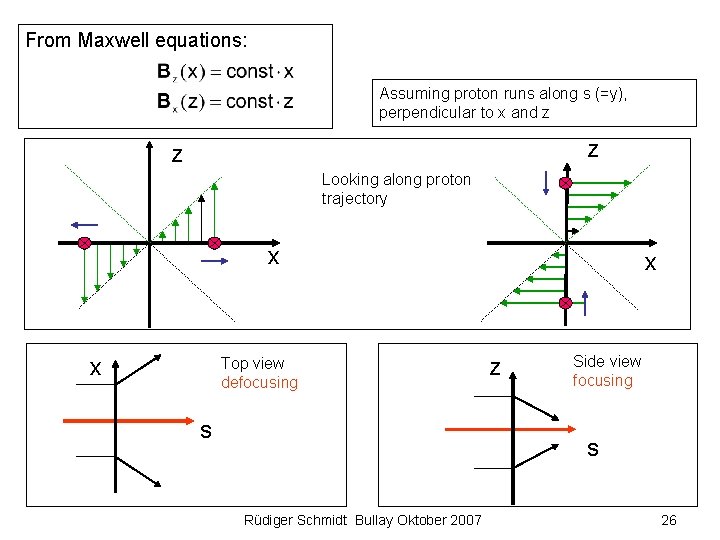
From Maxwell equations: Assuming proton runs along s (=y), perpendicular to x and z z z Looking along proton trajectory x x Top view defocusing s x z Side view focusing s Rüdiger Schmidt Bullay Oktober 2007 26
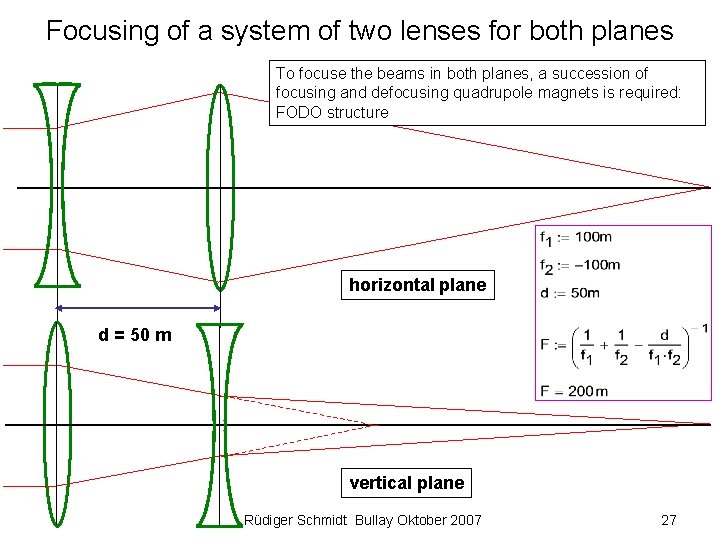
Focusing of a system of two lenses for both planes To focuse the beams in both planes, a succession of focusing and defocusing quadrupole magnets is required: FODO structure horizontal plane d = 50 m vertical plane Rüdiger Schmidt Bullay Oktober 2007 27
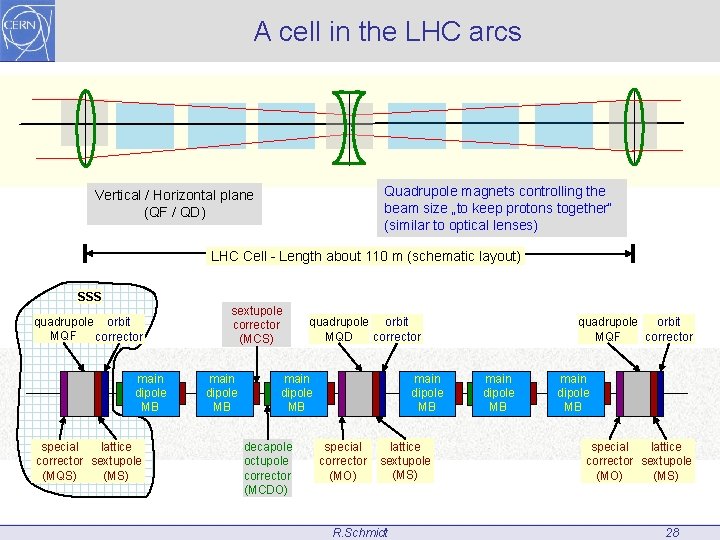
A cell in the LHC arcs Quadrupole magnets controlling the beam size „to keep protons together“ (similar to optical lenses) Vertical / Horizontal plane (QF / QD) LHC Cell - Length about 110 m (schematic layout) SSS quadrupole orbit MQF corrector main dipole MB special lattice corrector sextupole (MQS) (MS) sextupole corrector (MCS) main dipole MB quadrupole orbit MQD corrector main dipole MB decapole octupole corrector (MCDO) main dipole MB special corrector (MO) lattice sextupole (MS) R. Schmidt quadrupole orbit MQF corrector main dipole MB special lattice corrector sextupole (MO) (MS) 28
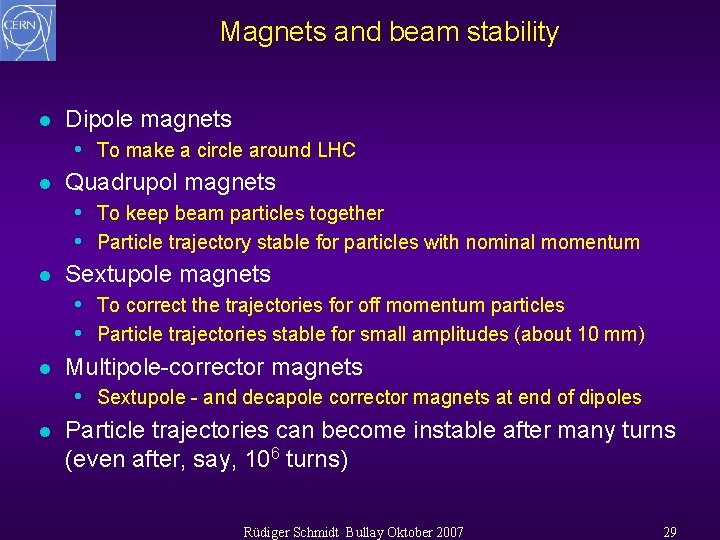
Magnets and beam stability l l l Dipole magnets • To make a circle around LHC Quadrupol magnets • To keep beam particles together • Particle trajectory stable for particles with nominal momentum Sextupole magnets • To correct the trajectories for off momentum particles • Particle trajectories stable for small amplitudes (about 10 mm) Multipole-corrector magnets • Sextupole - and decapole corrector magnets at end of dipoles Particle trajectories can become instable after many turns (even after, say, 106 turns) Rüdiger Schmidt Bullay Oktober 2007 29
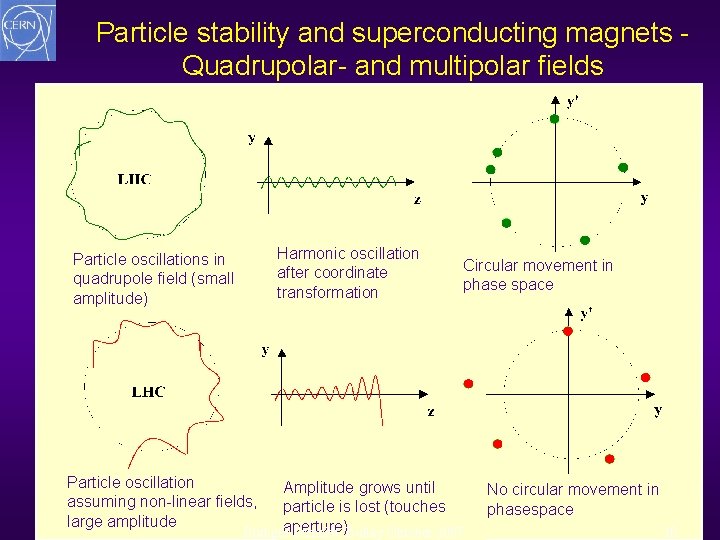
Particle stability and superconducting magnets Quadrupolar- and multipolar fields Harmonic oscillation after coordinate transformation Particle oscillations in quadrupole field (small amplitude) Particle oscillation assuming non-linear fields, large amplitude Circular movement in phase space Amplitude grows until particle is lost (touches Rüdigeraperture) Schmidt Bullay Oktober 2007 No circular movement in phasespace 30
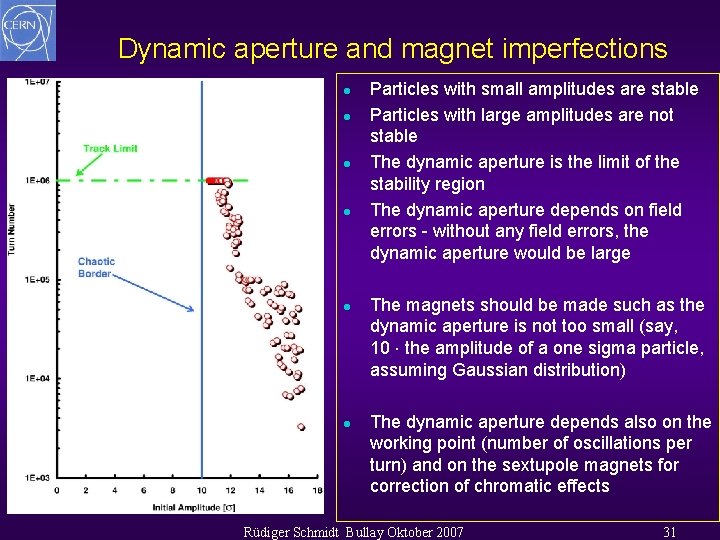
Dynamic aperture and magnet imperfections l l l Particles with small amplitudes are stable Particles with large amplitudes are not stable The dynamic aperture is the limit of the stability region The dynamic aperture depends on field errors - without any field errors, the dynamic aperture would be large The magnets should be made such as the dynamic aperture is not too small (say, 10 the amplitude of a one sigma particle, assuming Gaussian distribution) The dynamic aperture depends also on the working point (number of oscillations per turn) and on the sextupole magnets for correction of chromatic effects Rüdiger Schmidt Bullay Oktober 2007 31
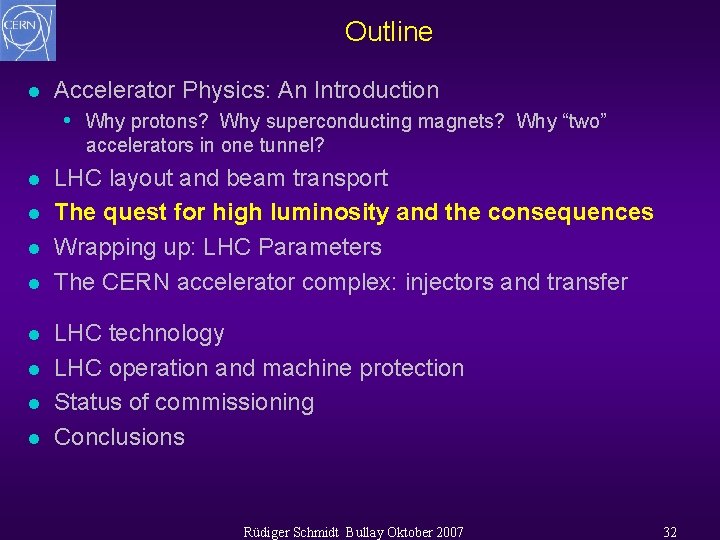
Outline l Accelerator Physics: An Introduction • Why protons? Why superconducting magnets? Why “two” accelerators in one tunnel? l l l l LHC layout and beam transport The quest for high luminosity and the consequences Wrapping up: LHC Parameters The CERN accelerator complex: injectors and transfer LHC technology LHC operation and machine protection Status of commissioning Conclusions Rüdiger Schmidt Bullay Oktober 2007 32
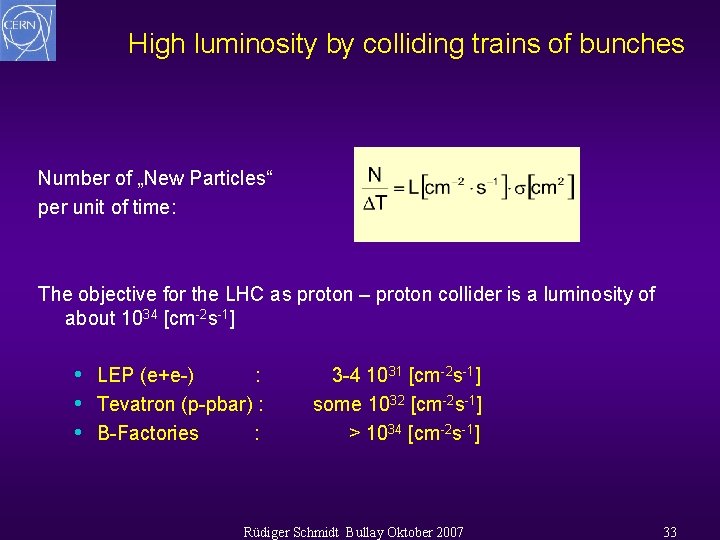
High luminosity by colliding trains of bunches Number of „New Particles“ per unit of time: The objective for the LHC as proton – proton collider is a luminosity of about 1034 [cm-2 s-1] • LEP (e+e-) : • Tevatron (p-pbar) : • B-Factories : 3 -4 1031 [cm-2 s-1] some 1032 [cm-2 s-1] > 1034 [cm-2 s-1] Rüdiger Schmidt Bullay Oktober 2007 33
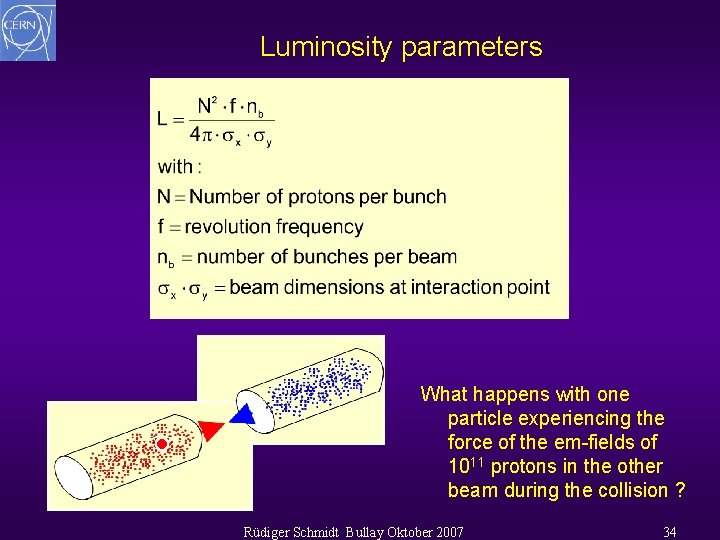
Luminosity parameters What happens with one particle experiencing the force of the em-fields of 1011 protons in the other beam during the collision ? Rüdiger Schmidt Bullay Oktober 2007 34
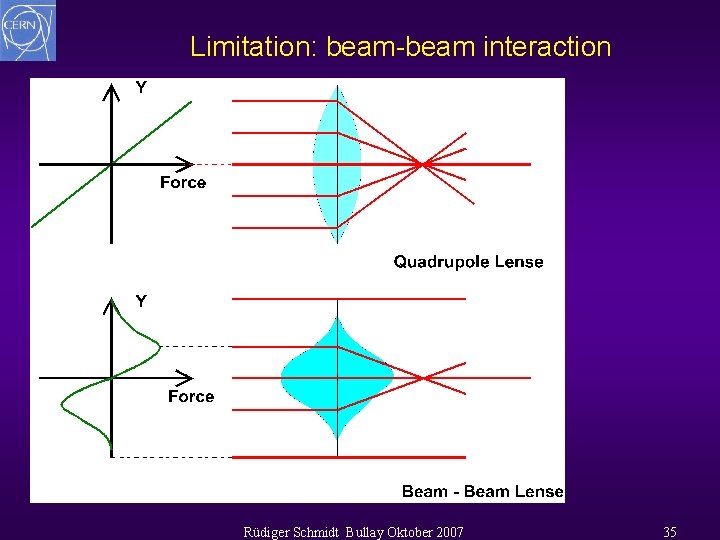
Limitation: beam-beam interaction Rüdiger Schmidt Bullay Oktober 2007 35
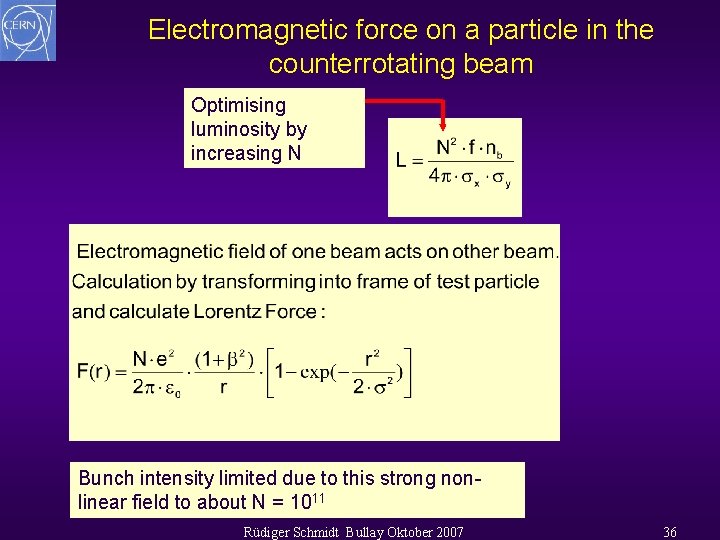
Electromagnetic force on a particle in the counterrotating beam Optimising luminosity by increasing N Bunch intensity limited due to this strong nonlinear field to about N = 1011 Rüdiger Schmidt Bullay Oktober 2007 36
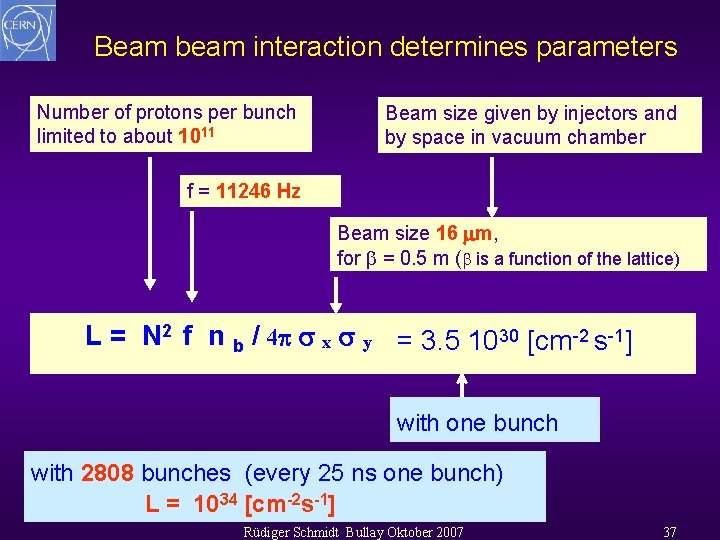
Beam beam interaction determines parameters Number of protons per bunch limited to about 1011 Beam size given by injectors and by space in vacuum chamber f = 11246 Hz Beam size 16 m, for = 0. 5 m ( is a function of the lattice) L = N 2 f n b / 4 p s x s y = 3. 5 1030 [cm-2 s-1] with one bunch with 2808 bunches (every 25 ns one bunch) L = 1034 [cm-2 s-1] Rüdiger Schmidt Bullay Oktober 2007 37
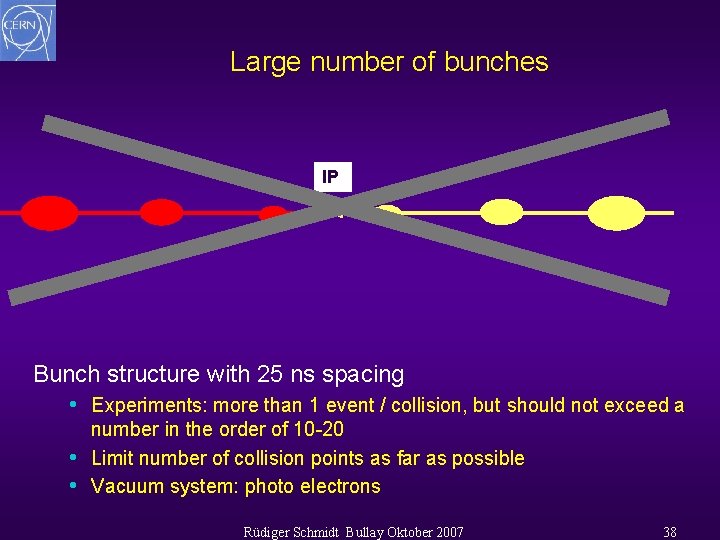
Large number of bunches IP Bunch structure with 25 ns spacing • Experiments: more than 1 event / collision, but should not exceed a • • number in the order of 10 -20 Limit number of collision points as far as possible Vacuum system: photo electrons Rüdiger Schmidt Bullay Oktober 2007 38
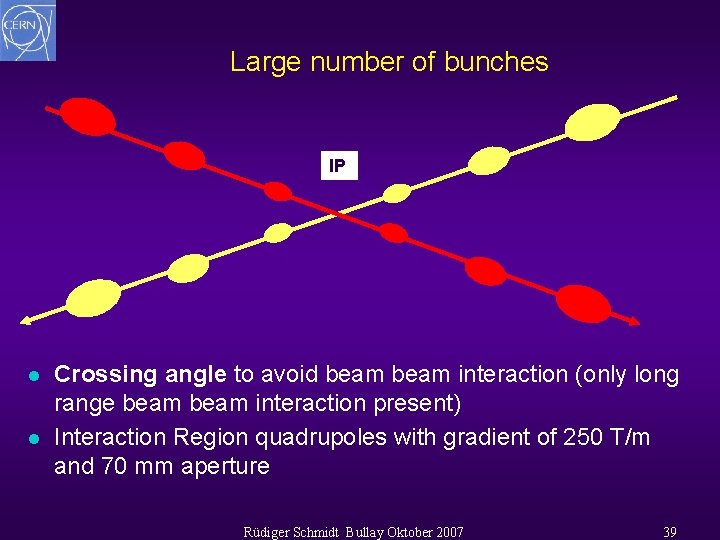
Large number of bunches IP l l Crossing angle to avoid beam interaction (only long range beam interaction present) Interaction Region quadrupoles with gradient of 250 T/m and 70 mm aperture Rüdiger Schmidt Bullay Oktober 2007 39
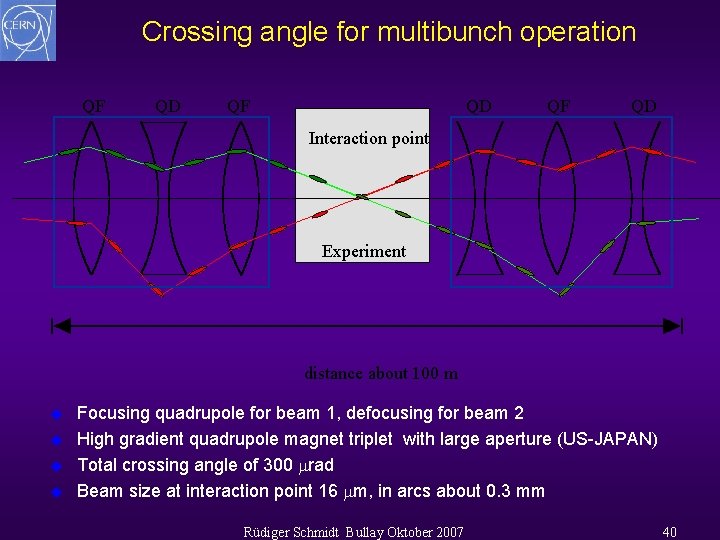
Crossing angle for multibunch operation QF QD Interaction point Experiment distance about 100 m u u Focusing quadrupole for beam 1, defocusing for beam 2 High gradient quadrupole magnet triplet with large aperture (US-JAPAN) Total crossing angle of 300 mrad Beam size at interaction point 16 mm, in arcs about 0. 3 mm Rüdiger Schmidt Bullay Oktober 2007 40
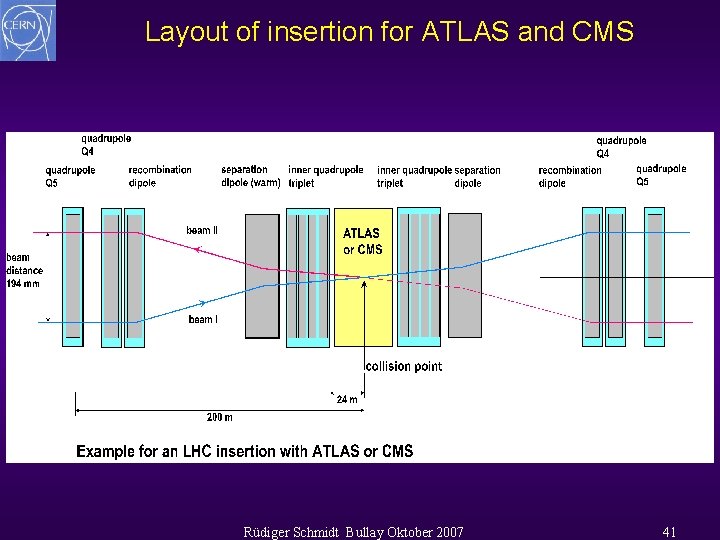
Layout of insertion for ATLAS and CMS Rüdiger Schmidt Bullay Oktober 2007 41
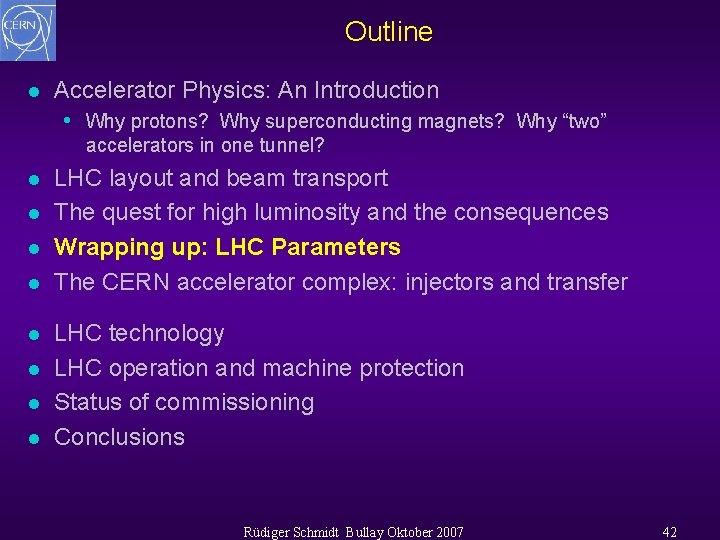
Outline l Accelerator Physics: An Introduction • Why protons? Why superconducting magnets? Why “two” accelerators in one tunnel? l l l l LHC layout and beam transport The quest for high luminosity and the consequences Wrapping up: LHC Parameters The CERN accelerator complex: injectors and transfer LHC technology LHC operation and machine protection Status of commissioning Conclusions Rüdiger Schmidt Bullay Oktober 2007 42
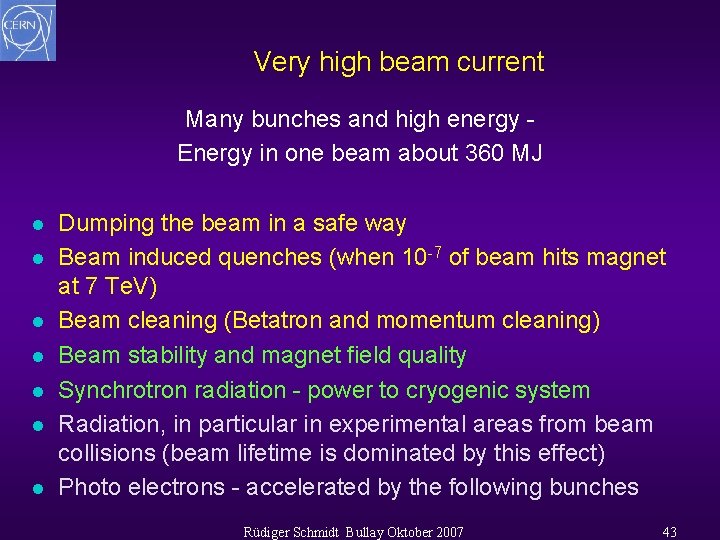
Very high beam current Many bunches and high energy Energy in one beam about 360 MJ l l l l Dumping the beam in a safe way Beam induced quenches (when 10 -7 of beam hits magnet at 7 Te. V) Beam cleaning (Betatron and momentum cleaning) Beam stability and magnet field quality Synchrotron radiation - power to cryogenic system Radiation, in particular in experimental areas from beam collisions (beam lifetime is dominated by this effect) Photo electrons - accelerated by the following bunches Rüdiger Schmidt Bullay Oktober 2007 43
![Challenges Energy stored in the beam MJ One beam nominal intensity corresponds to an Challenges: Energy stored in the beam [MJ] One beam, nominal intensity (corresponds to an](https://slidetodoc.com/presentation_image_h2/f8af96389038b62d47681788c18d4c7e/image-44.jpg)
Challenges: Energy stored in the beam [MJ] One beam, nominal intensity (corresponds to an energy that melts 500 kg of copper) x 200 x 10000 courtesy R. Assmann Momentum [Ge. V/c] Transverse energy. Rüdiger density: even a factor 1000 larger Schmidt Bullay Oktoberof 2007 44
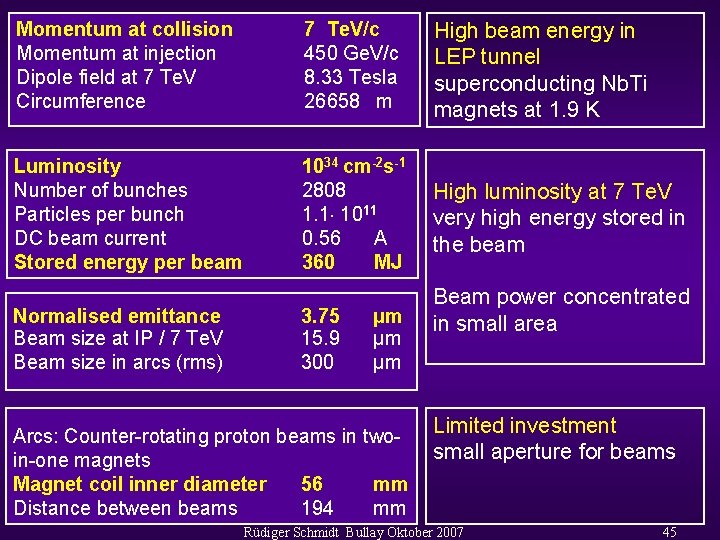
Momentum at collision Momentum at injection Dipole field at 7 Te. V Circumference 7 Te. V/c 450 Ge. V/c 8. 33 Tesla 26658 m High beam energy in LEP tunnel superconducting Nb. Ti magnets at 1. 9 K Luminosity Number of bunches Particles per bunch DC beam current Stored energy per beam 1034 cm-2 s-1 2808 1. 1 1011 0. 56 A 360 MJ High luminosity at 7 Te. V very high energy stored in the beam Normalised emittance Beam size at IP / 7 Te. V Beam size in arcs (rms) 3. 75 15. 9 300 µm µm µm Arcs: Counter-rotating proton beams in twoin-one magnets Magnet coil inner diameter 56 mm Distance between beams 194 mm Beam power concentrated in small area Limited investment small aperture for beams Rüdiger Schmidt Bullay Oktober 2007 45
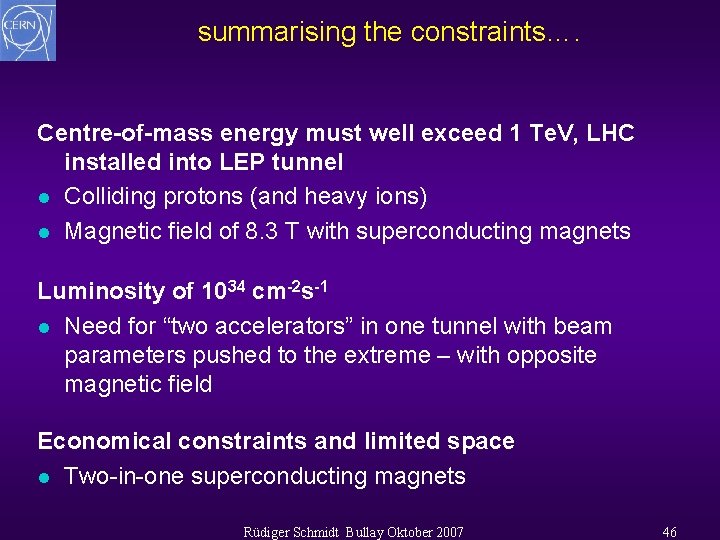
summarising the constraints…. Centre-of-mass energy must well exceed 1 Te. V, LHC installed into LEP tunnel l Colliding protons (and heavy ions) l Magnetic field of 8. 3 T with superconducting magnets Luminosity of 1034 cm-2 s-1 l Need for “two accelerators” in one tunnel with beam parameters pushed to the extreme – with opposite magnetic field Economical constraints and limited space l Two-in-one superconducting magnets Rüdiger Schmidt Bullay Oktober 2007 46
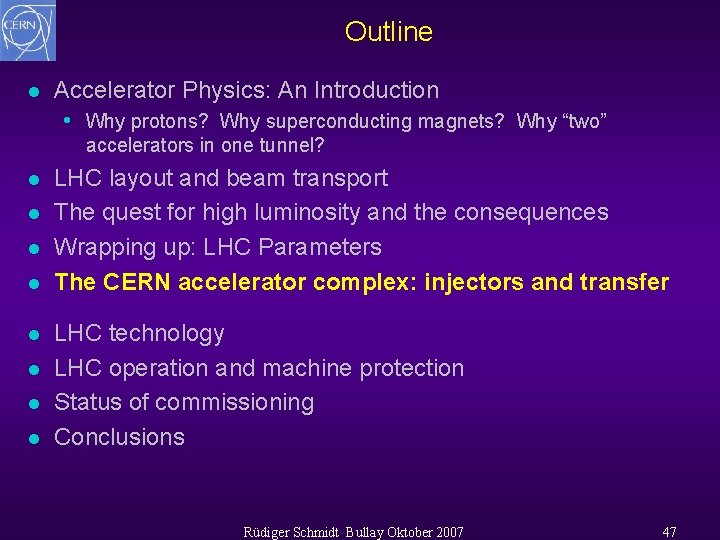
Outline l Accelerator Physics: An Introduction • Why protons? Why superconducting magnets? Why “two” accelerators in one tunnel? l l l l LHC layout and beam transport The quest for high luminosity and the consequences Wrapping up: LHC Parameters The CERN accelerator complex: injectors and transfer LHC technology LHC operation and machine protection Status of commissioning Conclusions Rüdiger Schmidt Bullay Oktober 2007 47
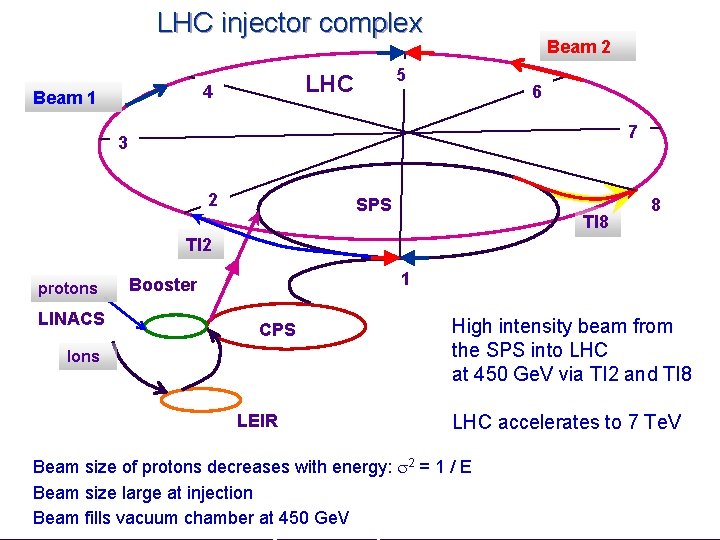
LHC injector complex 5 LHC 4 Beam 1 Beam 2 6 7 3 2 SPS TI 8 8 TI 2 protons LINACS 1 Booster CPS Ions LEIR High intensity beam from the SPS into LHC at 450 Ge. V via TI 2 and TI 8 LHC accelerates to 7 Te. V Beam size of protons decreases with energy: 2 = 1 / E Beam size large at injection Beam fills vacuum chamber at 450 Ge. V Rüdiger Schmidt Bullay Oktober 2007 48
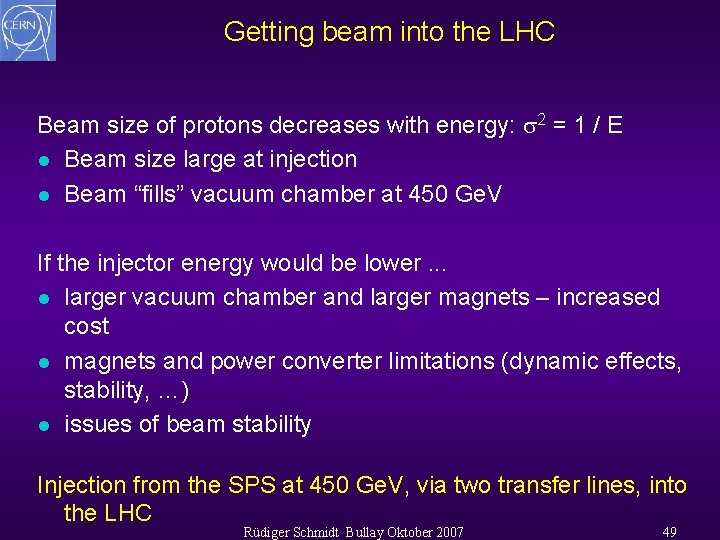
Getting beam into the LHC Beam size of protons decreases with energy: 2 = 1 / E l Beam size large at injection l Beam “fills” vacuum chamber at 450 Ge. V If the injector energy would be lower. . . l larger vacuum chamber and larger magnets – increased cost l magnets and power converter limitations (dynamic effects, stability, …) l issues of beam stability Injection from the SPS at 450 Ge. V, via two transfer lines, into the LHC Rüdiger Schmidt Bullay Oktober 2007 49
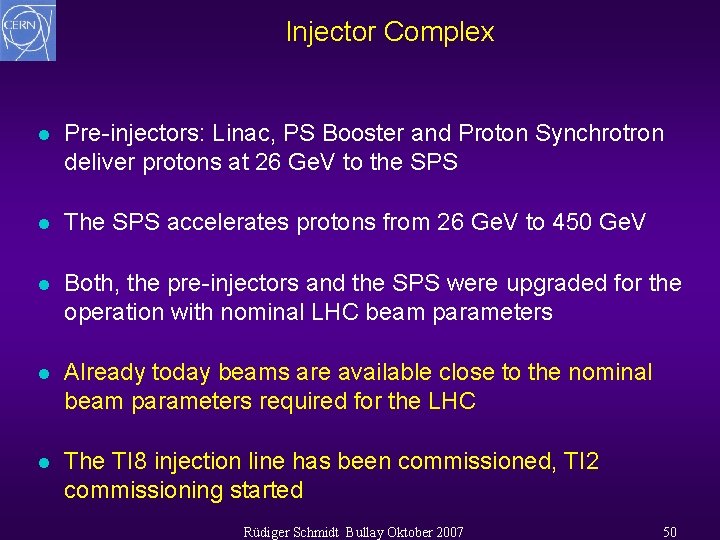
Injector Complex l Pre-injectors: Linac, PS Booster and Proton Synchrotron deliver protons at 26 Ge. V to the SPS l The SPS accelerates protons from 26 Ge. V to 450 Ge. V l Both, the pre-injectors and the SPS were upgraded for the operation with nominal LHC beam parameters l Already today beams are available close to the nominal beam parameters required for the LHC l The TI 8 injection line has been commissioned, TI 2 commissioning started Rüdiger Schmidt Bullay Oktober 2007 50
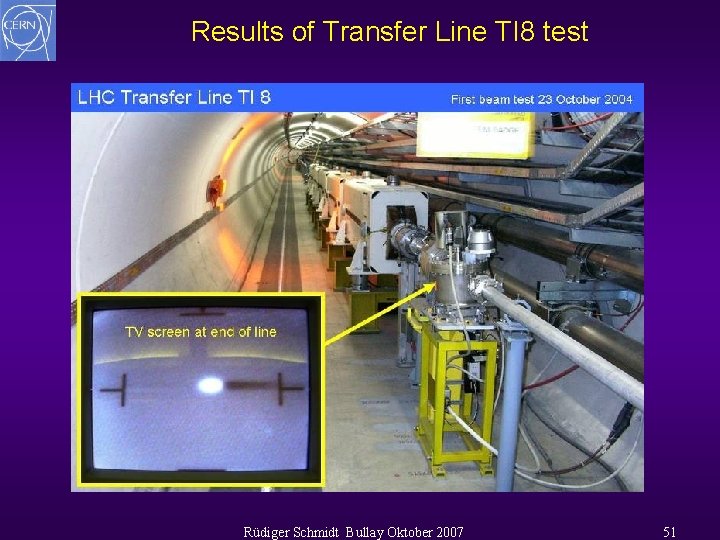
Results of Transfer Line TI 8 test Rüdiger Schmidt Bullay Oktober 2007 51
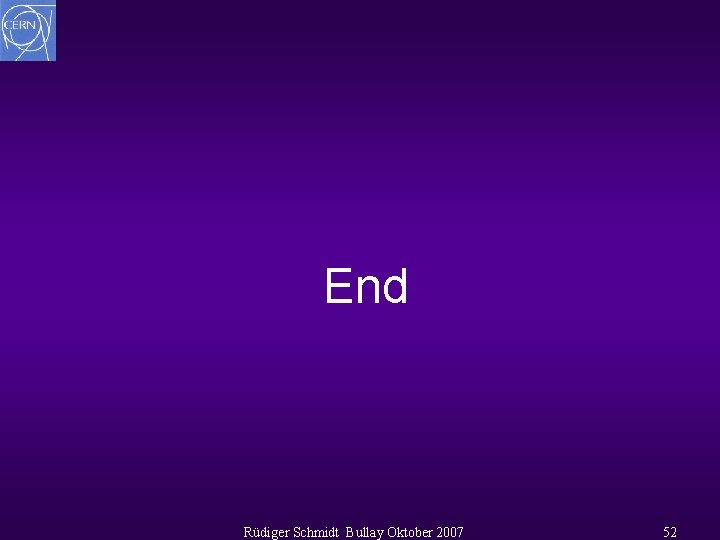
End Rüdiger Schmidt Bullay Oktober 2007 52
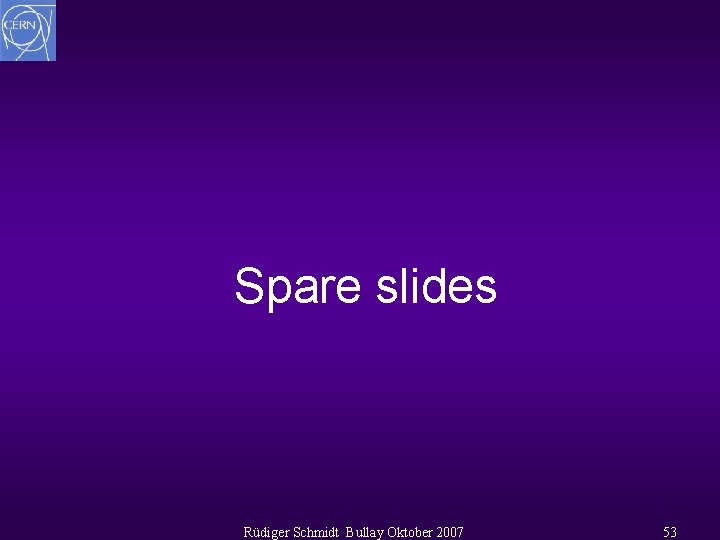
Spare slides Rüdiger Schmidt Bullay Oktober 2007 53
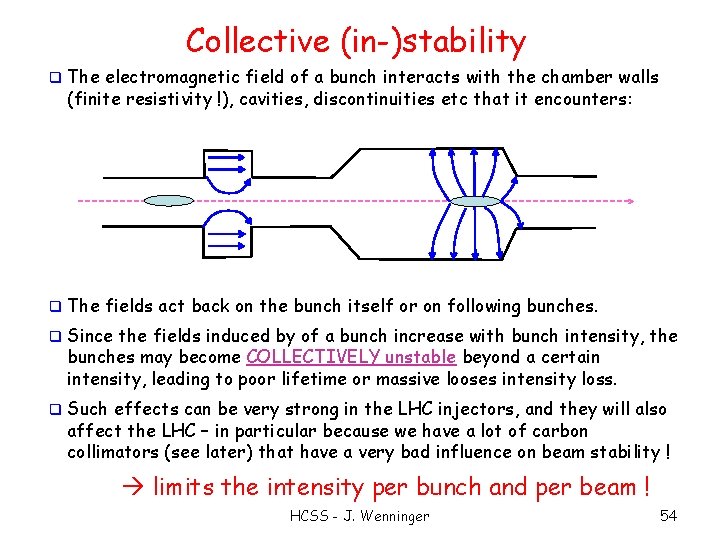
Collective (in-)stability q The electromagnetic field of a bunch interacts with the chamber walls (finite resistivity !), cavities, discontinuities etc that it encounters: q The fields act back on the bunch itself or on following bunches. q Since the fields induced by of a bunch increase with bunch intensity, the bunches may become COLLECTIVELY unstable beyond a certain intensity, leading to poor lifetime or massive looses intensity loss. q Such effects can be very strong in the LHC injectors, and they will also affect the LHC – in particular because we have a lot of carbon collimators (see later) that have a very bad influence on beam stability ! limits the intensity per bunch and per beam ! HCSS - J. Wenninger 54
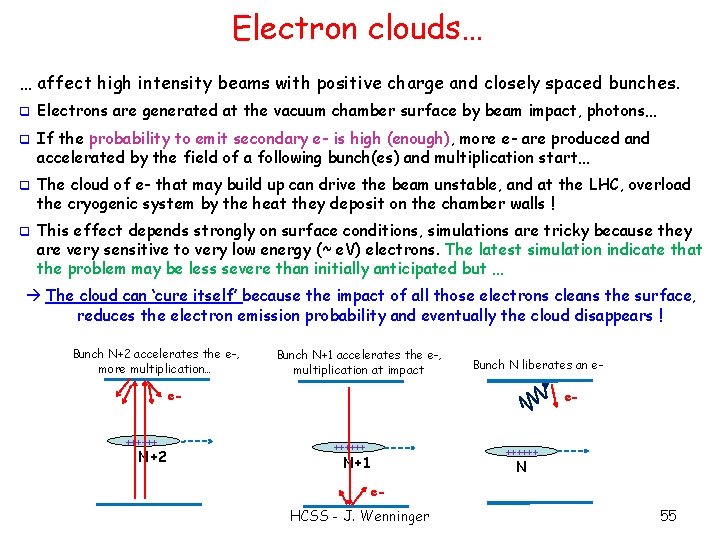
Electron clouds… … affect high intensity beams with positive charge and closely spaced bunches. q Electrons are generated at the vacuum chamber surface by beam impact, photons… q If the probability to emit secondary e- is high (enough), more e- are produced and accelerated by the field of a following bunch(es) and multiplication start… q The cloud of e- that may build up can drive the beam unstable, and at the LHC, overload the cryogenic system by the heat they deposit on the chamber walls ! q This effect depends strongly on surface conditions, simulations are tricky because they are very sensitive to very low energy (~ e. V) electrons. The latest simulation indicate that the problem may be less severe than initially anticipated but … The cloud can ‘cure itself’ because the impact of all those electrons cleans the surface, reduces the electron emission probability and eventually the cloud disappears ! Bunch N+2 accelerates the e-, more multiplication… Bunch N+1 accelerates the e-, multiplication at impact Bunch N liberates an e- e- ++++++ N+2 e- ++++++ N+1 ++++++ N e- HCSS - J. Wenninger 55
Fcc-ee
Burkhard schmidt cern
Burkhard schmidt cern
Hadron collider
Fcc collider
Particle collider
International linear collider
Fcc collider
Unity sphere collider
Bnl
Hadron collider
Muon collider
Ti8 schedule
Lhc quench
Lhc
Lhc performance workshop
Forum lhc
Kme copper
Hl-lhc schedule
"lhc"
Lhc filling scheme
Lhc budget
Lhc technical coordination
Lhc beam dump
Lhc intranet
Lhc logbook
Lhc plan
Damien lafarge
Lhc:8001692
Gant chart
Lhc schedule 2022
Hl-lhc schedule
Tevatron vs lhc
Forum lhc
Hl-lhc schedule
Lhc morning meeting
Lhc tantalizing new physics
Lhc beam dump
"lhc"
Lhc performance workshop
Arkani-hamed
Louisiana housing commission
Steam hel
Lhc performance workshop
Worldwide lhc computing grid
Gấu đi như thế nào
Thiếu nhi thế giới liên hoan
Fecboak
Một số thể thơ truyền thống
Thế nào là hệ số cao nhất
Frameset trong html5
Sơ đồ cơ thể người
Tư thế ngồi viết
Số nguyên tố là số gì
đặc điểm cơ thể của người tối cổ
Các châu lục và đại dương trên thế giới