The LHC collider project I Rdiger Schmidt CERN
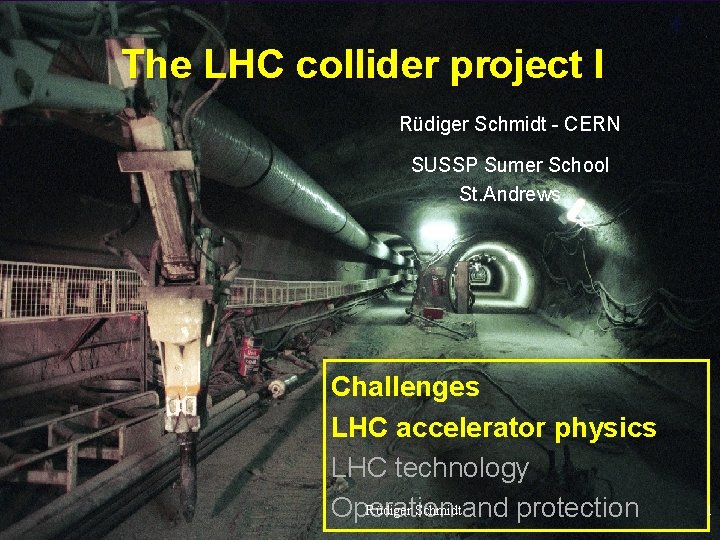
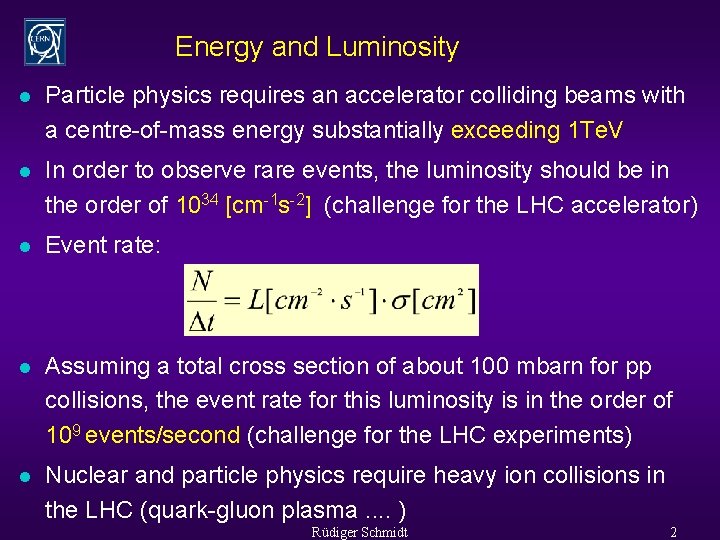
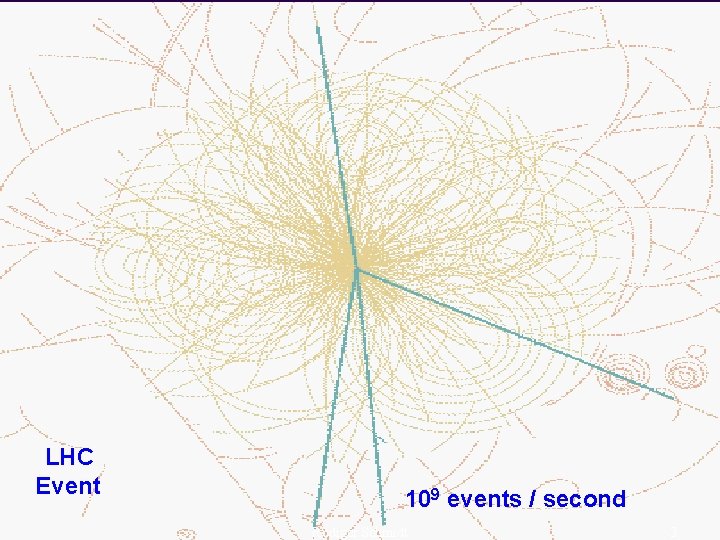
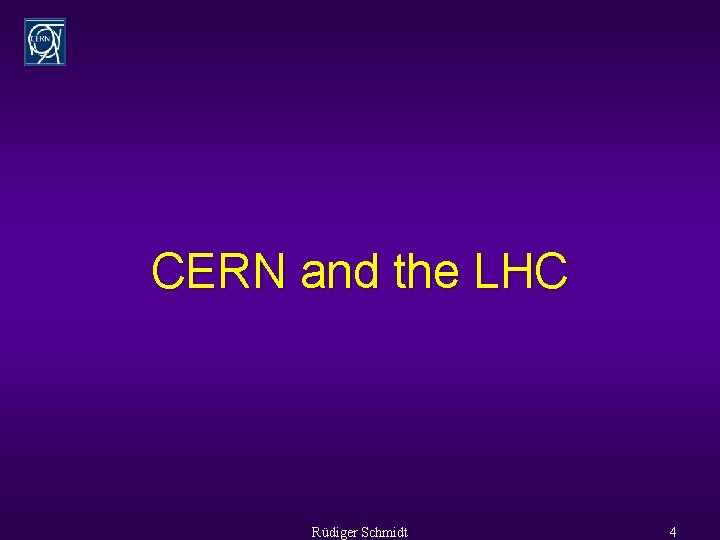
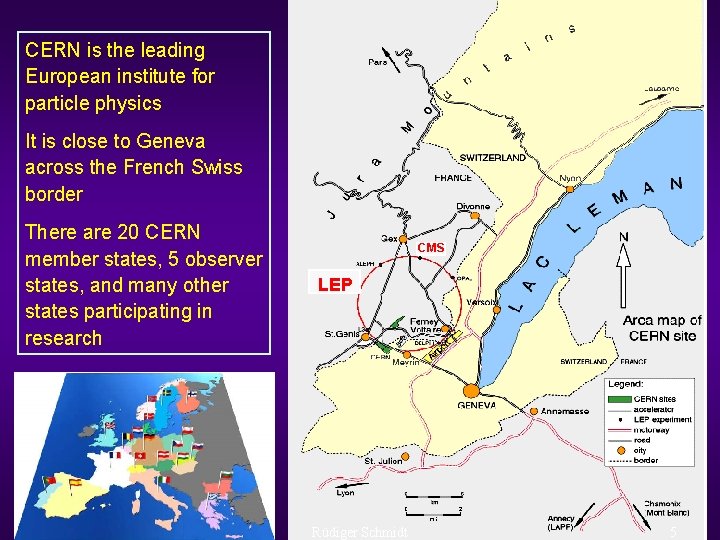
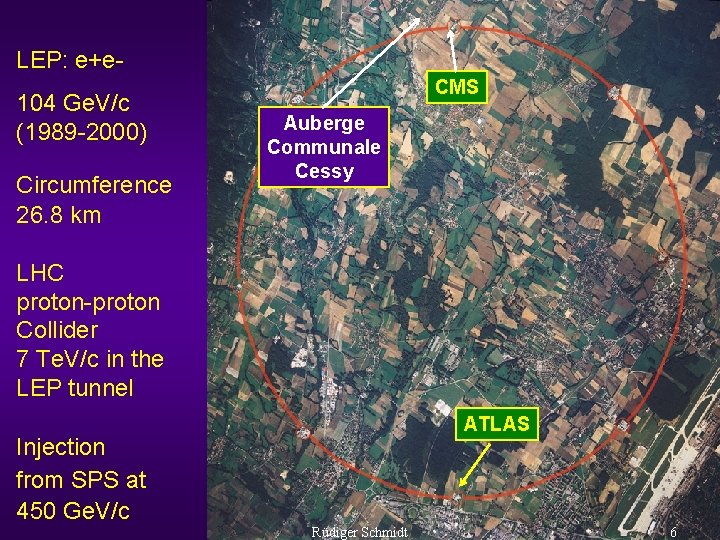
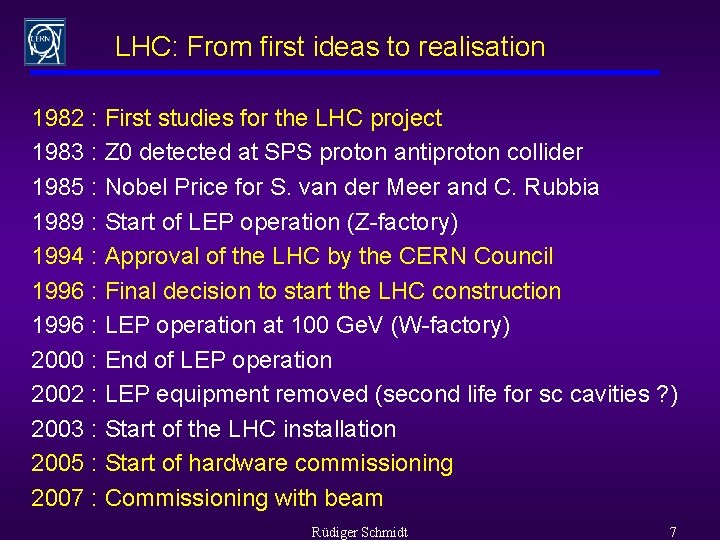
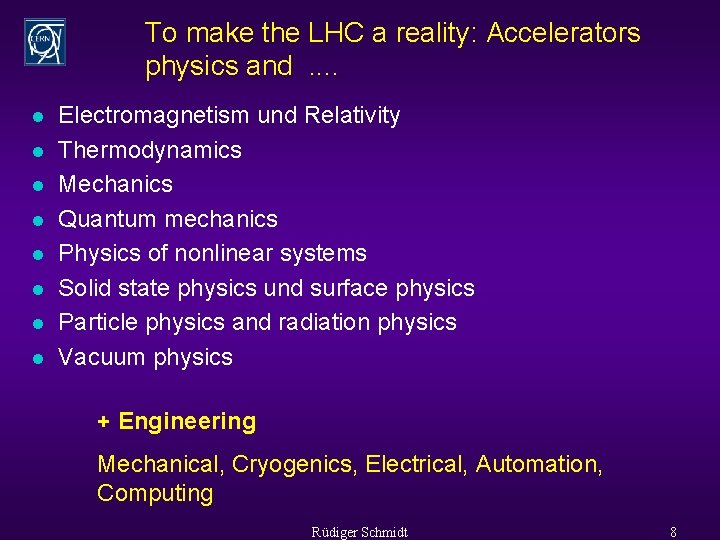
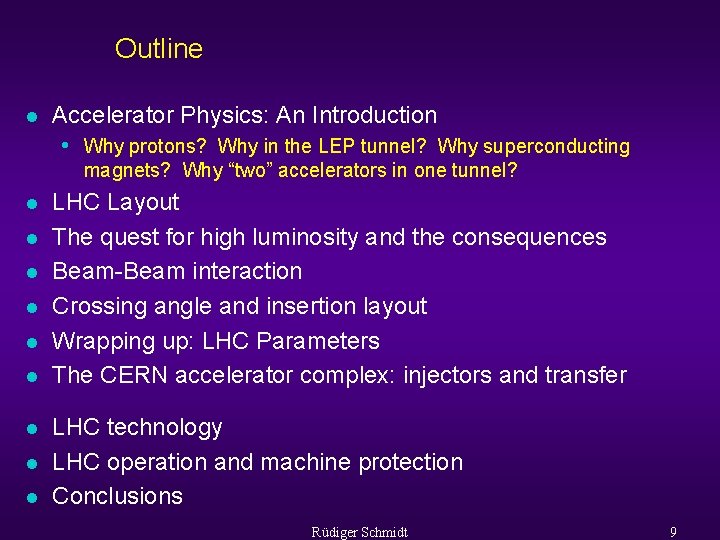
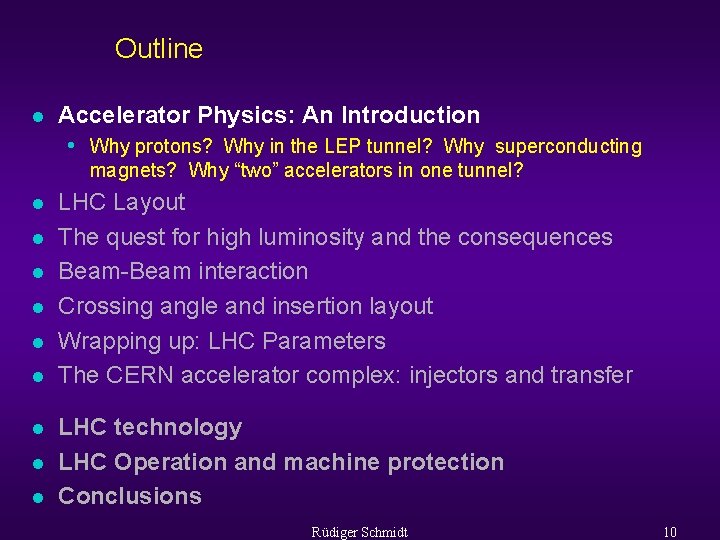
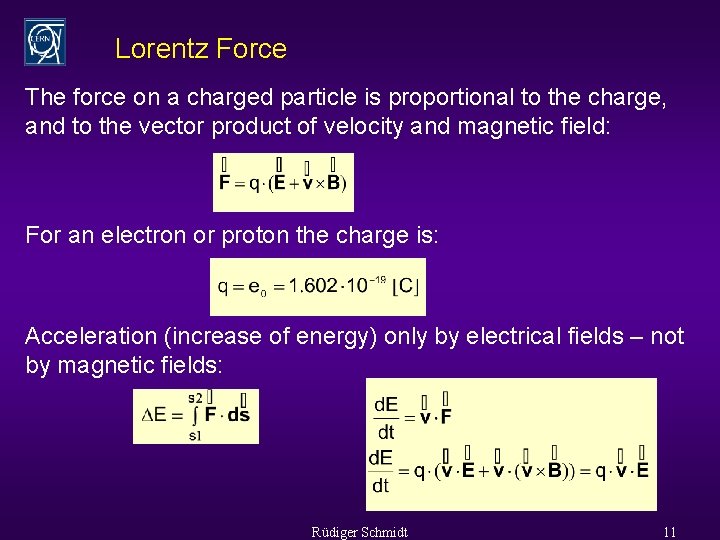
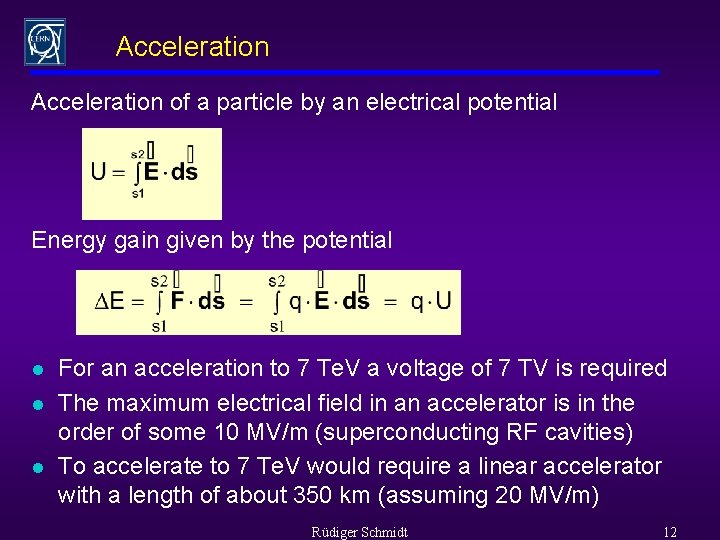
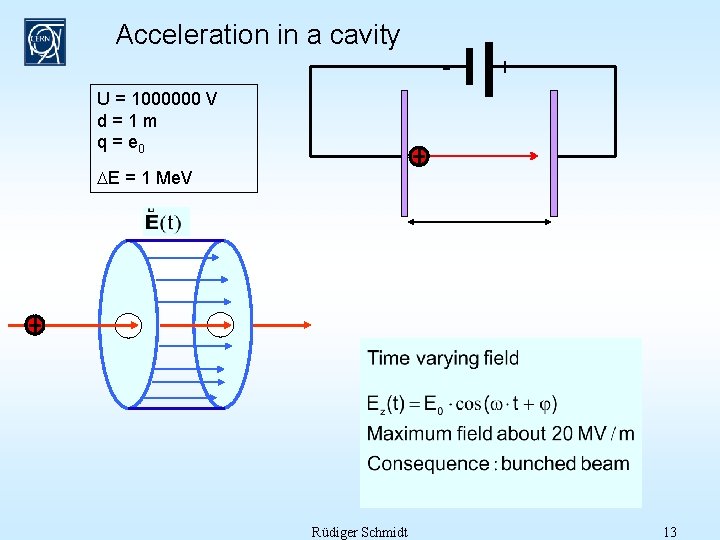
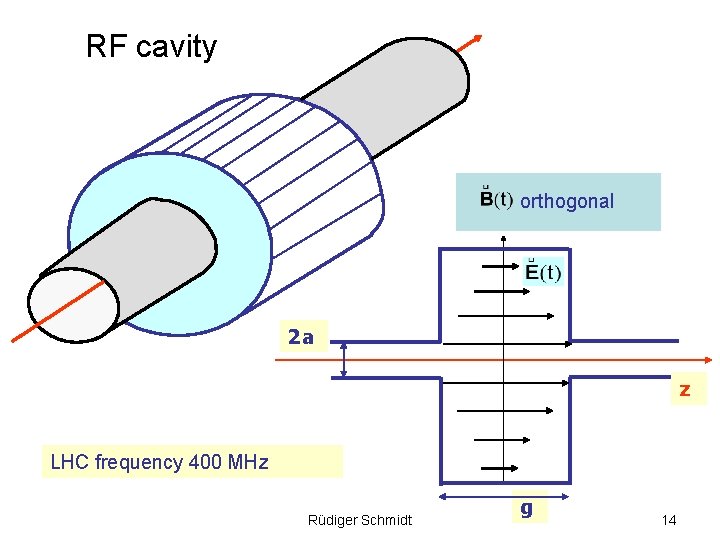
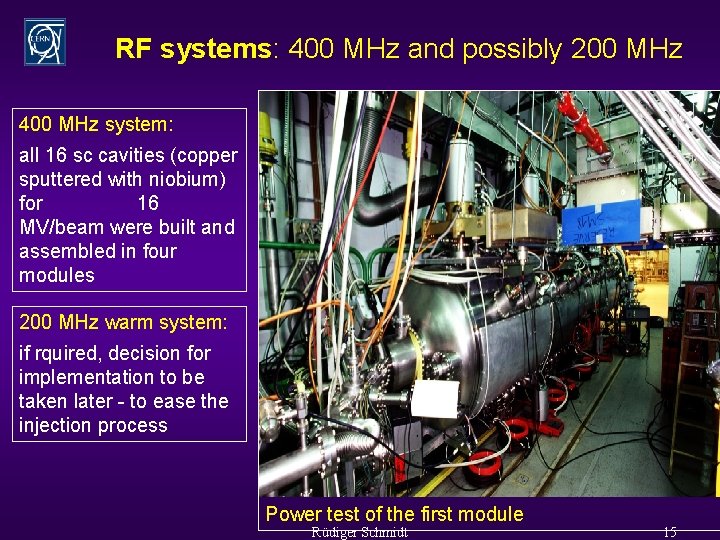
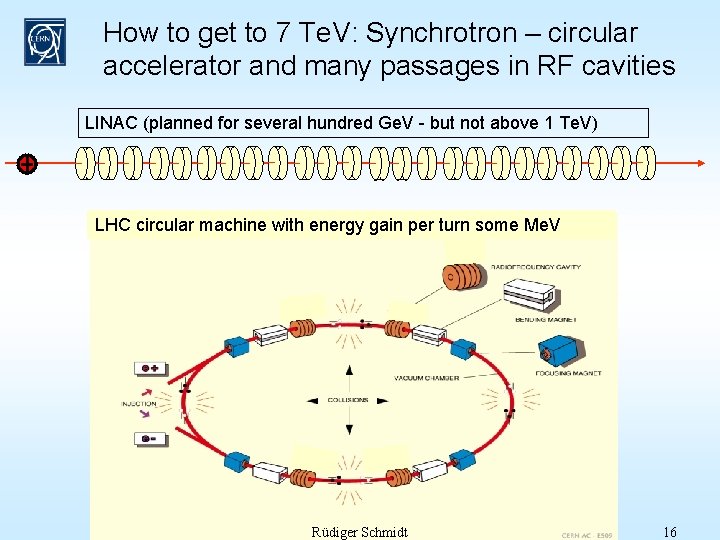
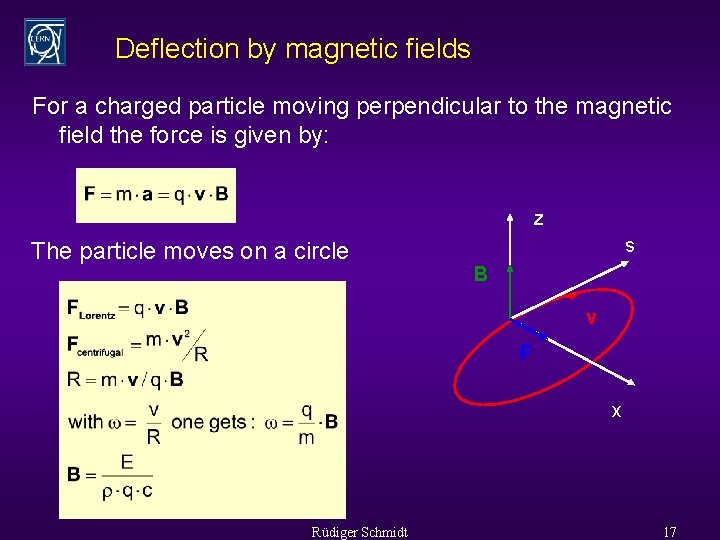
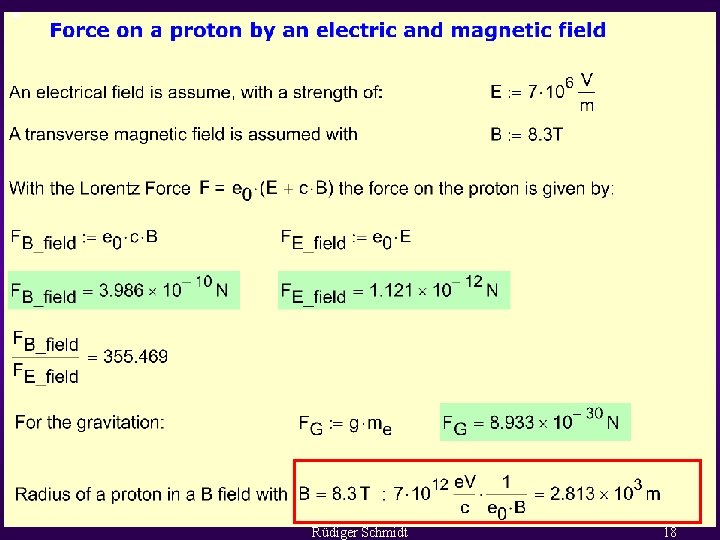
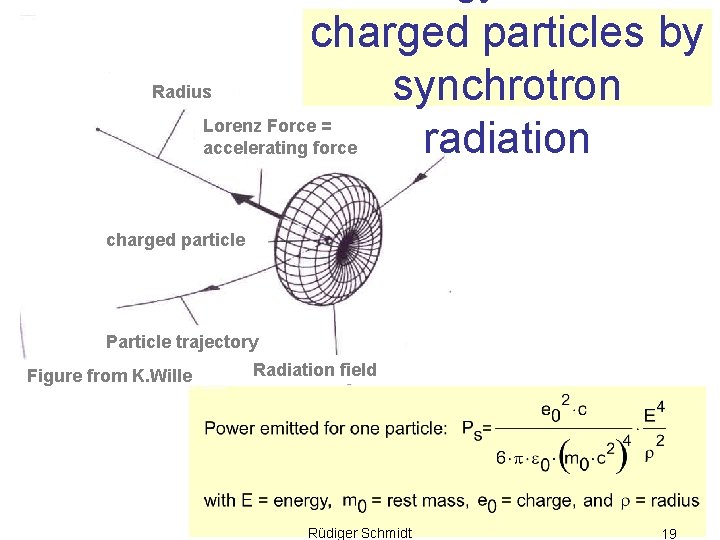
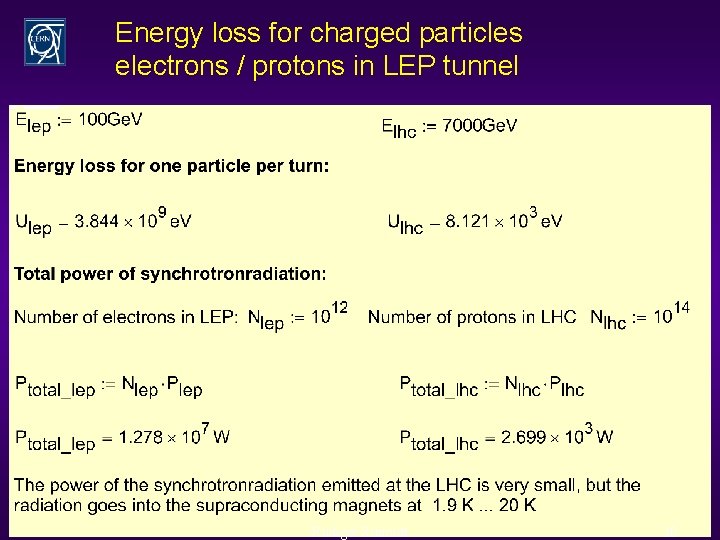
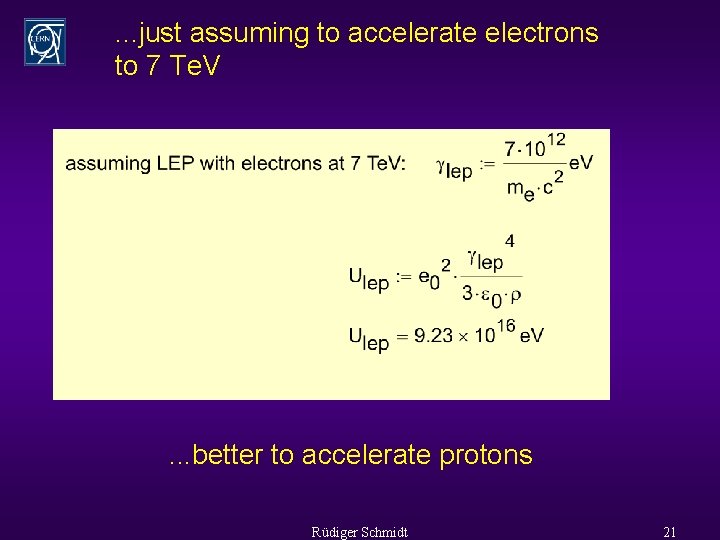
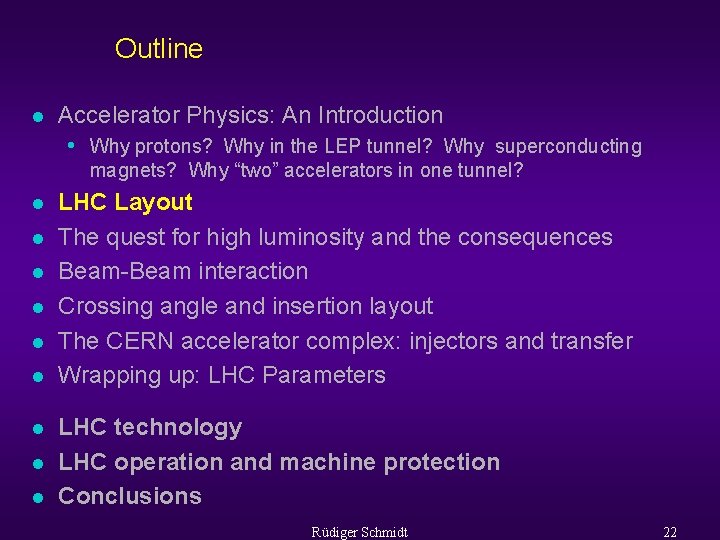
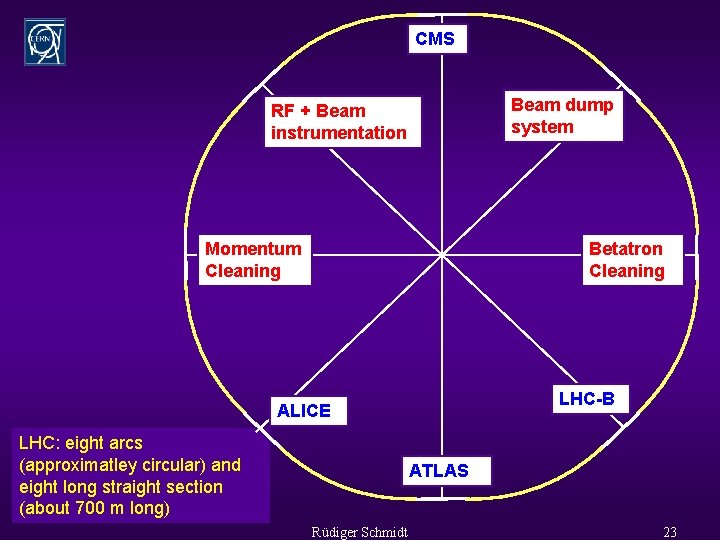
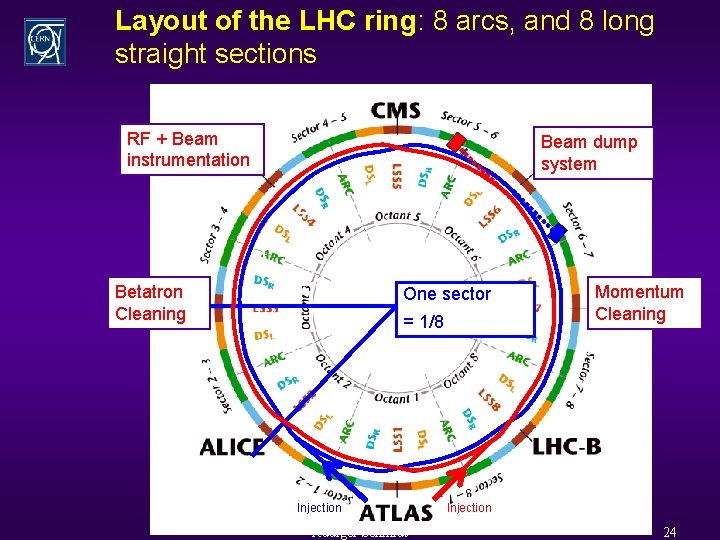
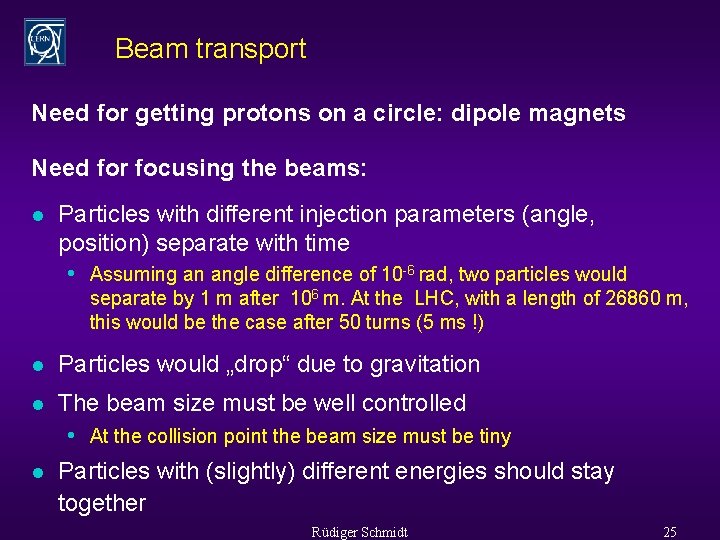
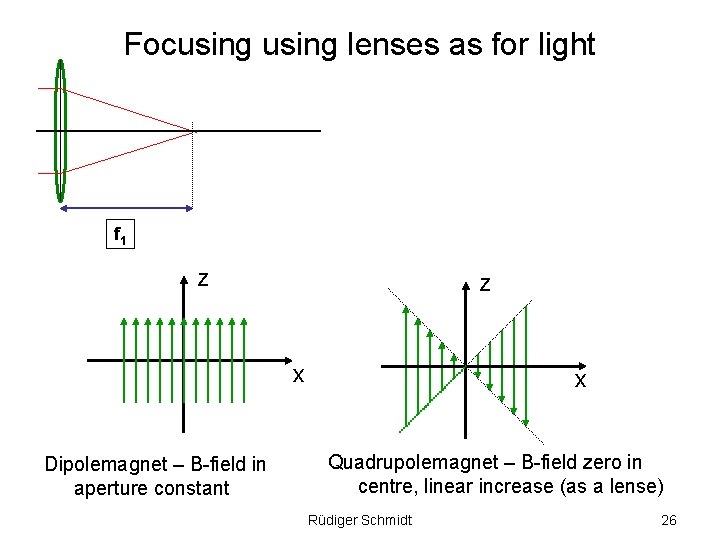
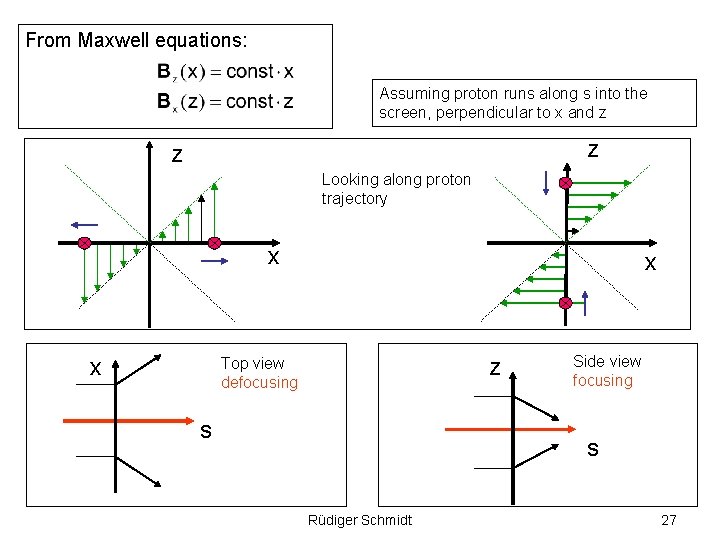
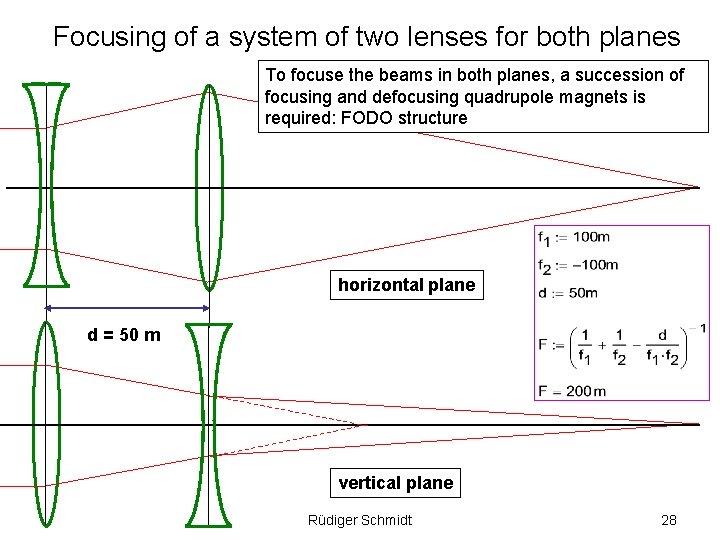
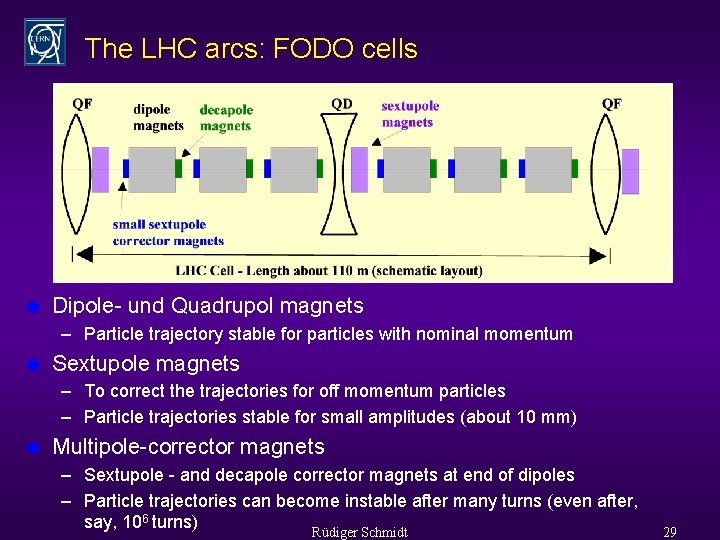
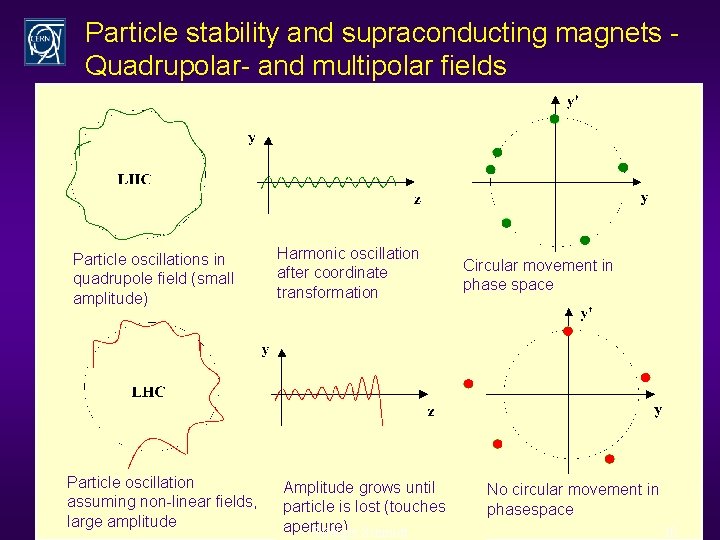
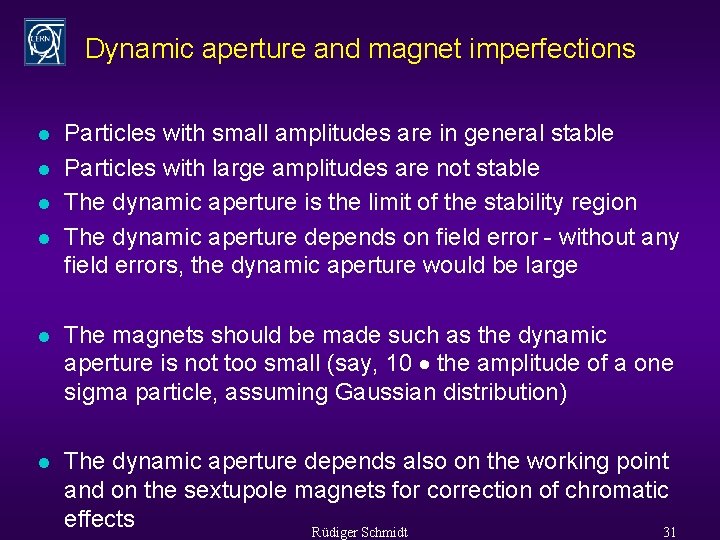
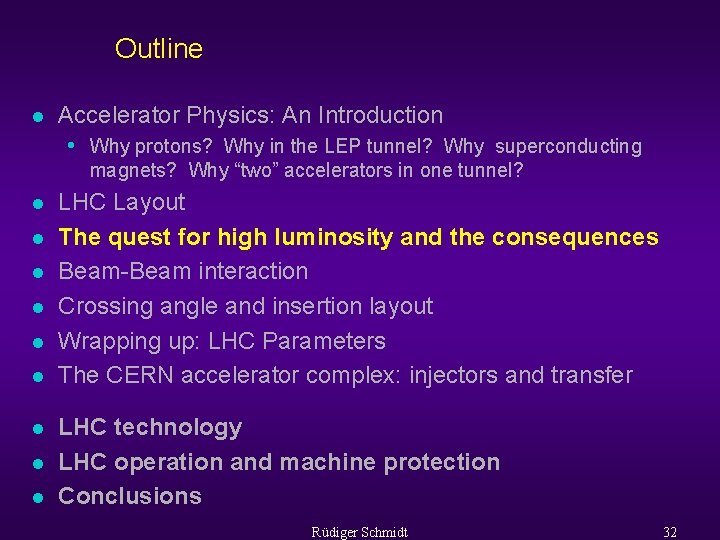
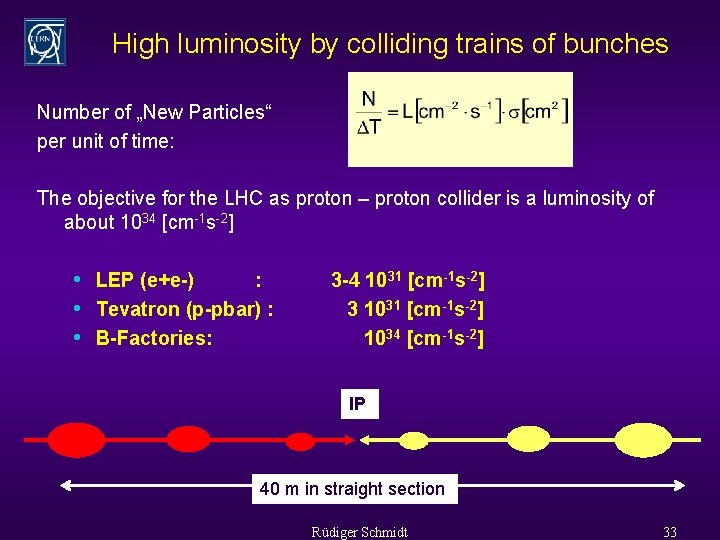
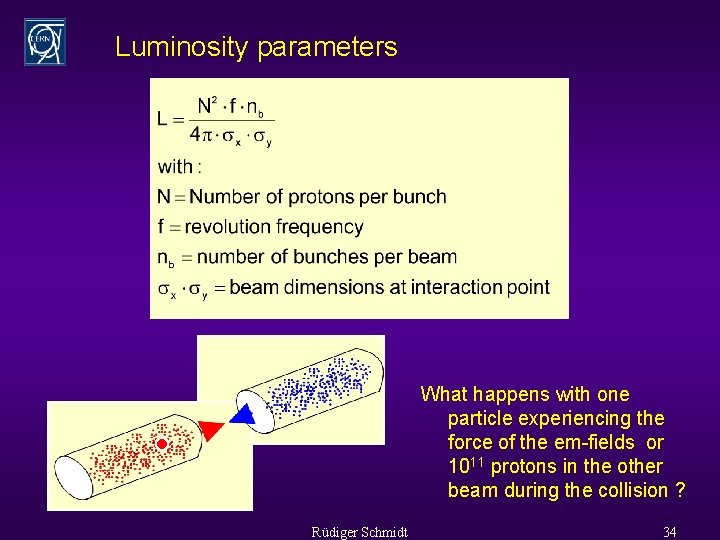
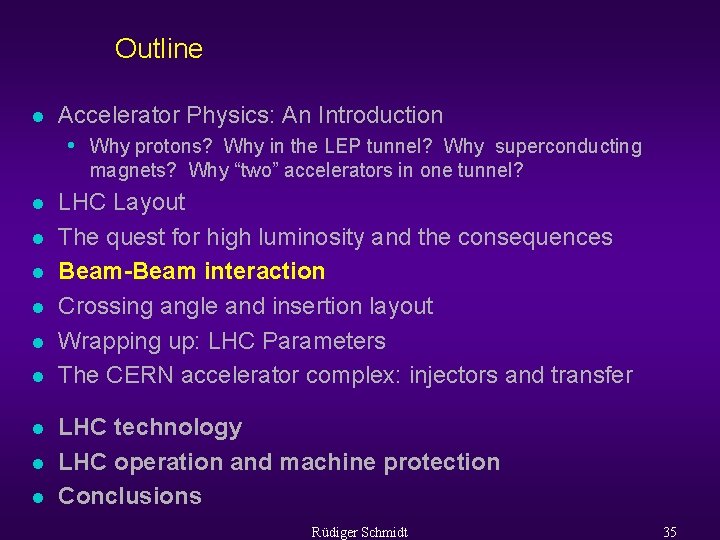
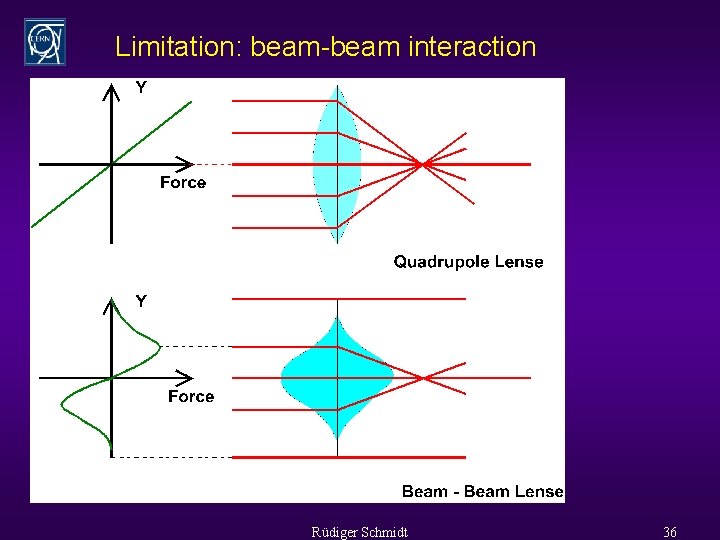
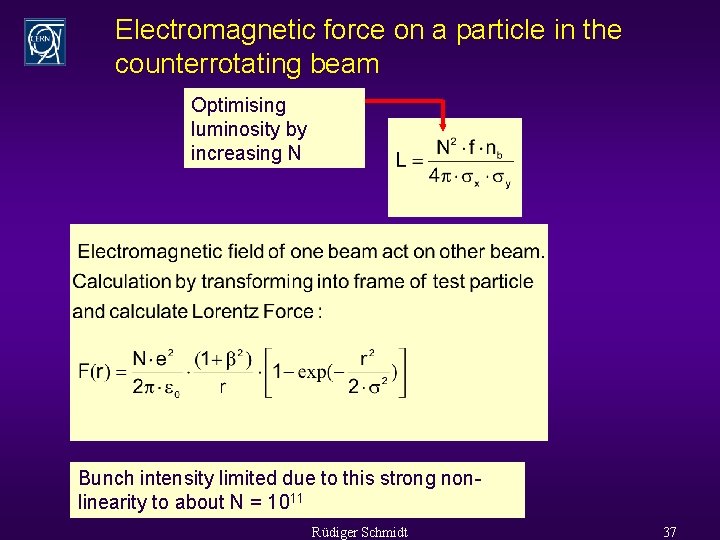
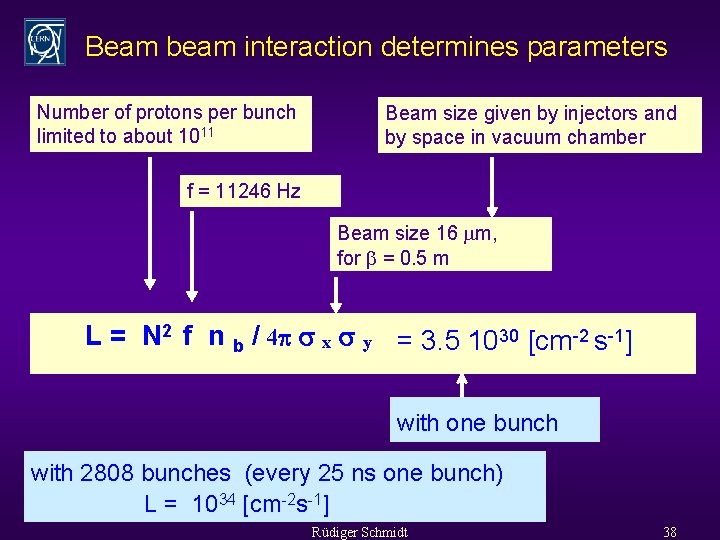
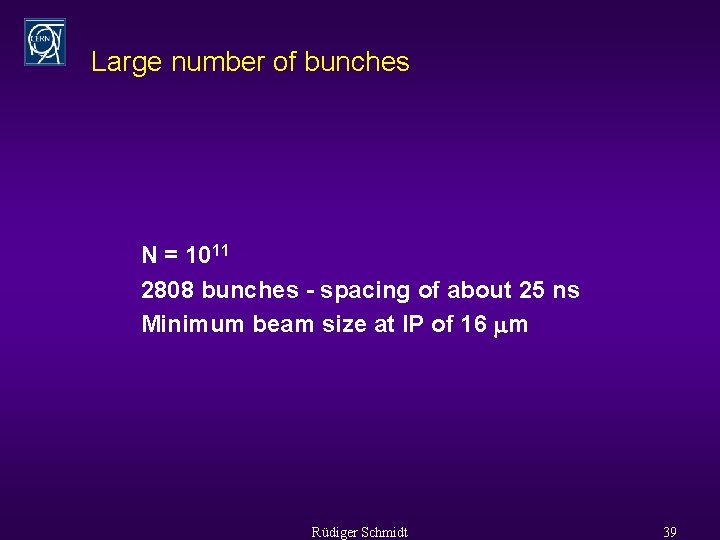
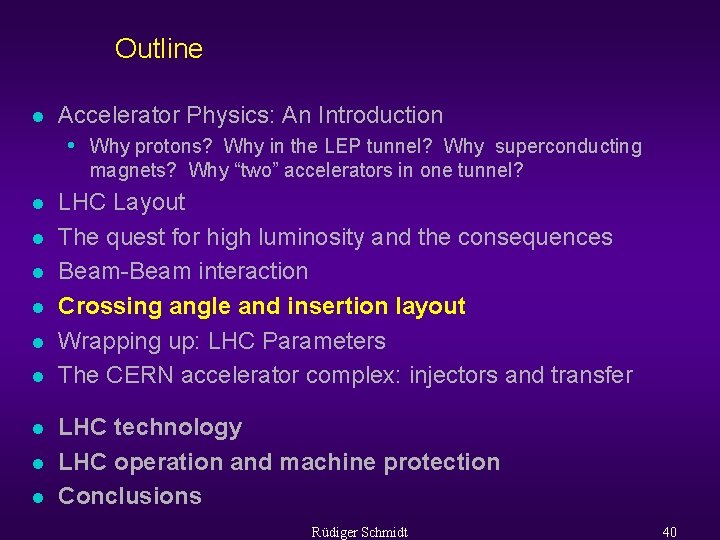
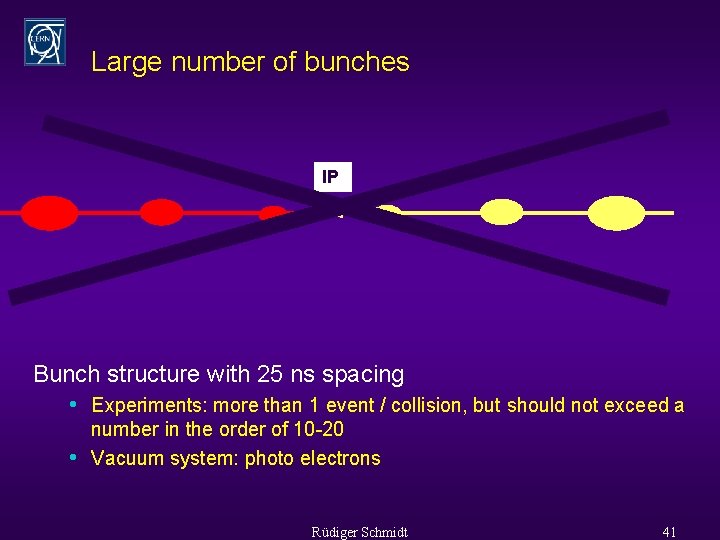
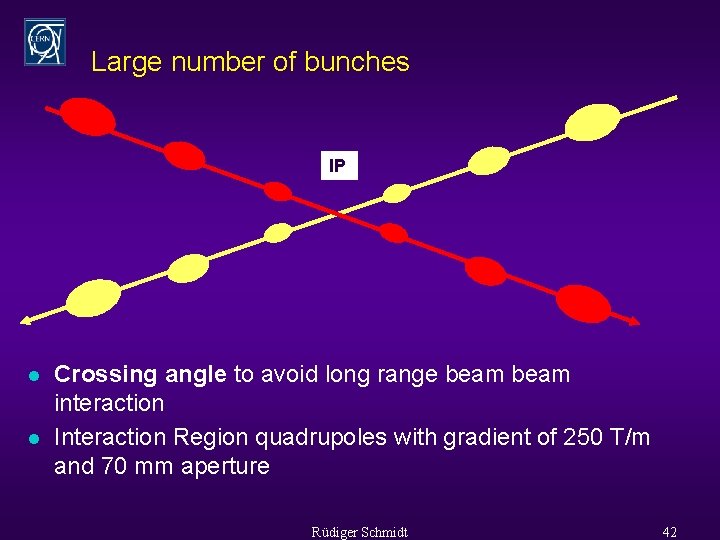
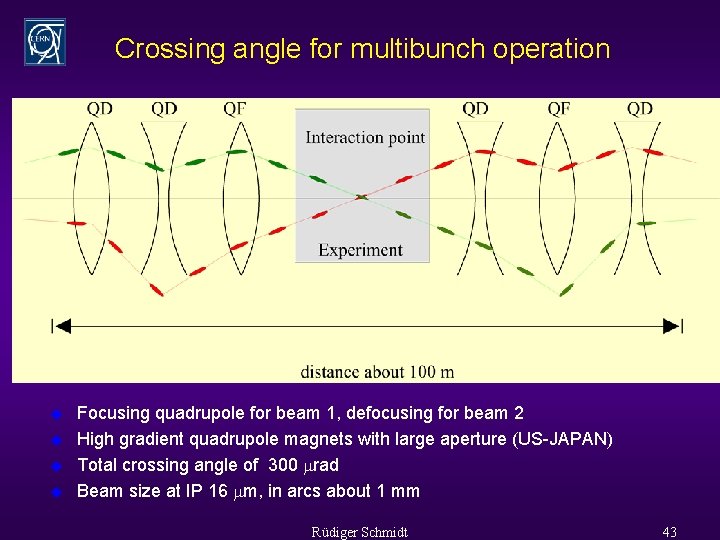
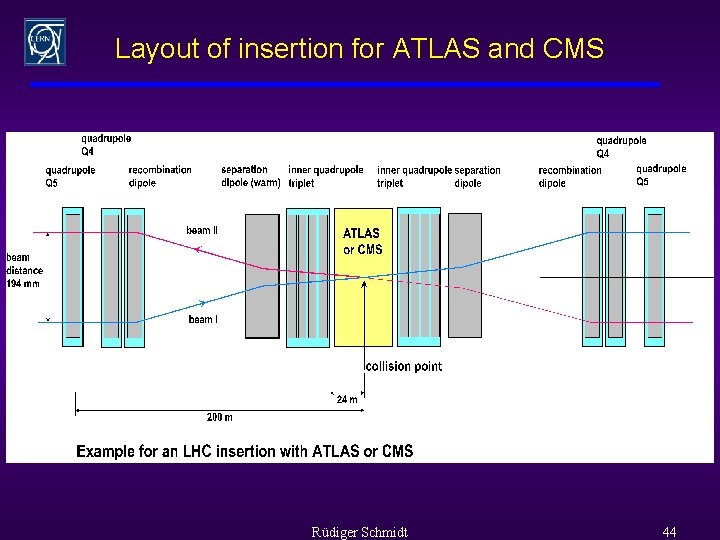
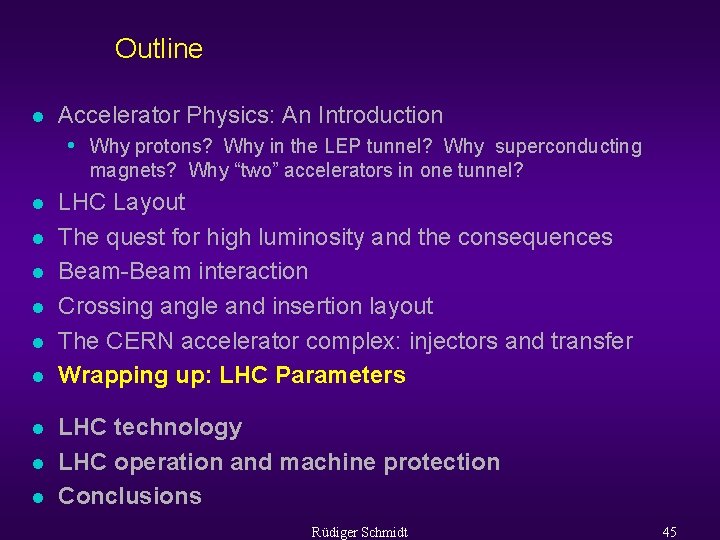
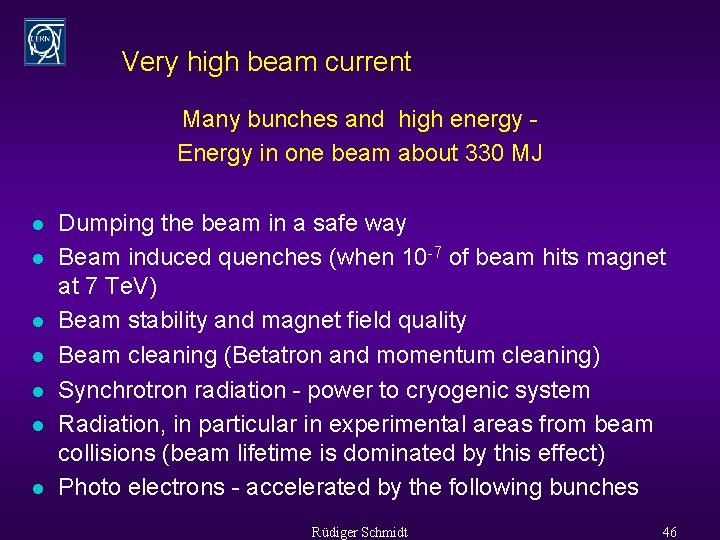
![Challenges: Energy stored in the beam [MJ] One beam, nominal intensity (corresponds to an Challenges: Energy stored in the beam [MJ] One beam, nominal intensity (corresponds to an](https://slidetodoc.com/presentation_image_h2/3eba6217c3402601657c9dcc9384af2e/image-47.jpg)
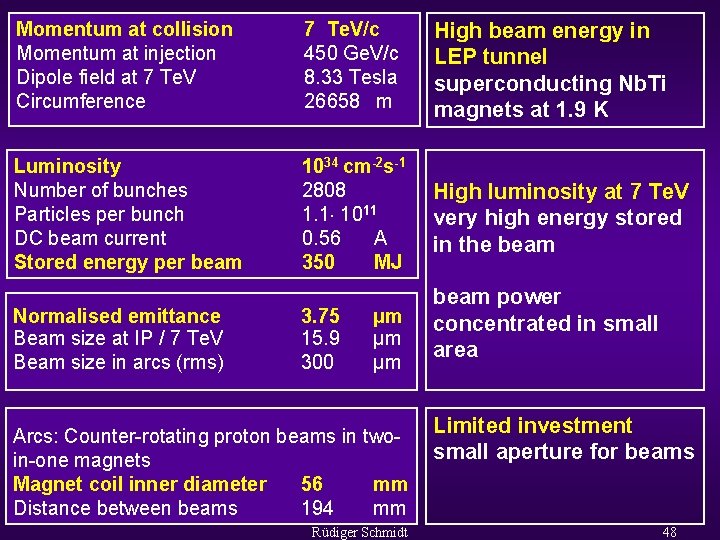
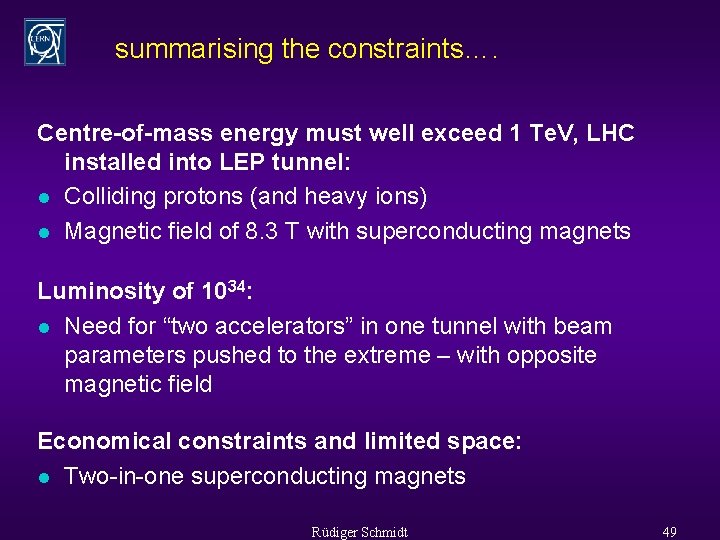
- Slides: 49
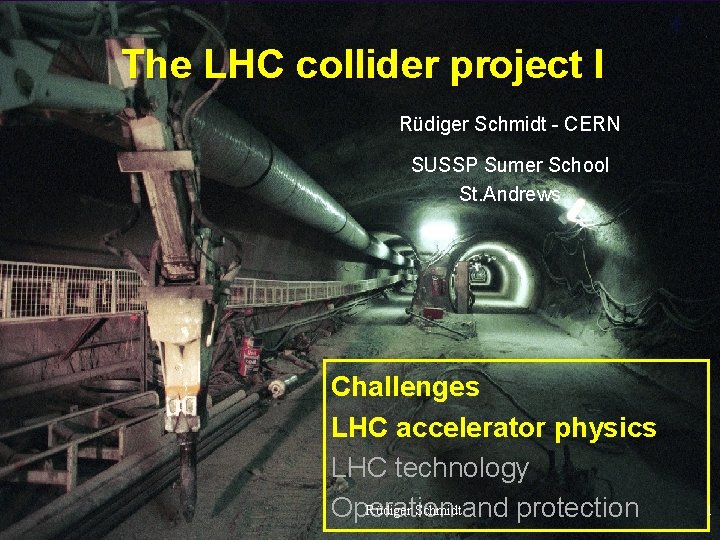
The LHC collider project I Rüdiger Schmidt - CERN SUSSP Sumer School St. Andrews Challenges LHC accelerator physics LHC technology Rüdiger Schmidt and protection Operation 1
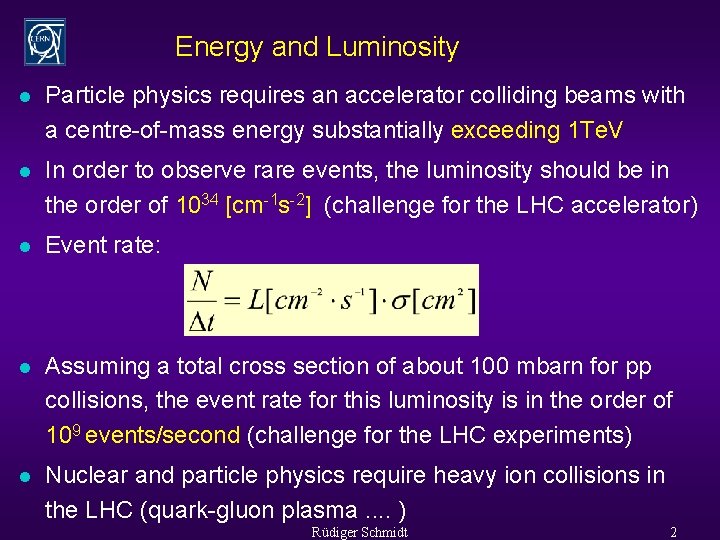
Energy and Luminosity l Particle physics requires an accelerator colliding beams with a centre-of-mass energy substantially exceeding 1 Te. V l In order to observe rare events, the luminosity should be in the order of 1034 [cm-1 s-2] (challenge for the LHC accelerator) l Event rate: l Assuming a total cross section of about 100 mbarn for pp collisions, the event rate for this luminosity is in the order of 109 events/second (challenge for the LHC experiments) l Nuclear and particle physics require heavy ion collisions in the LHC (quark-gluon plasma. . ) Rüdiger Schmidt 2
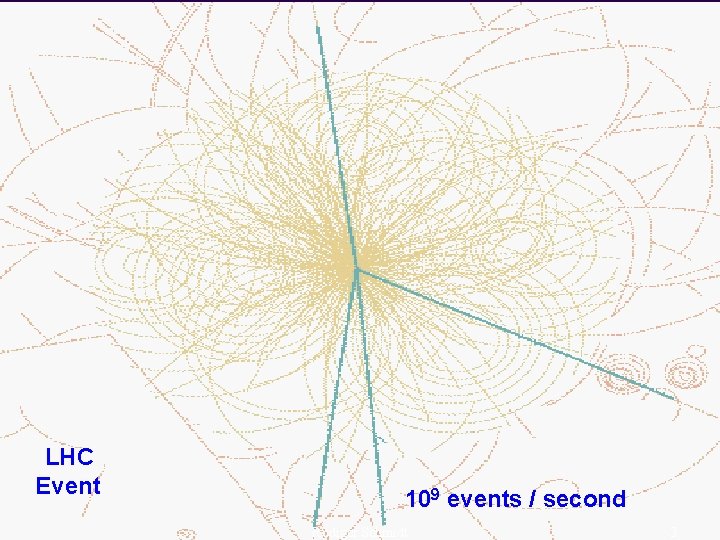
LHC Event 109 events / second Rüdiger Schmidt 3
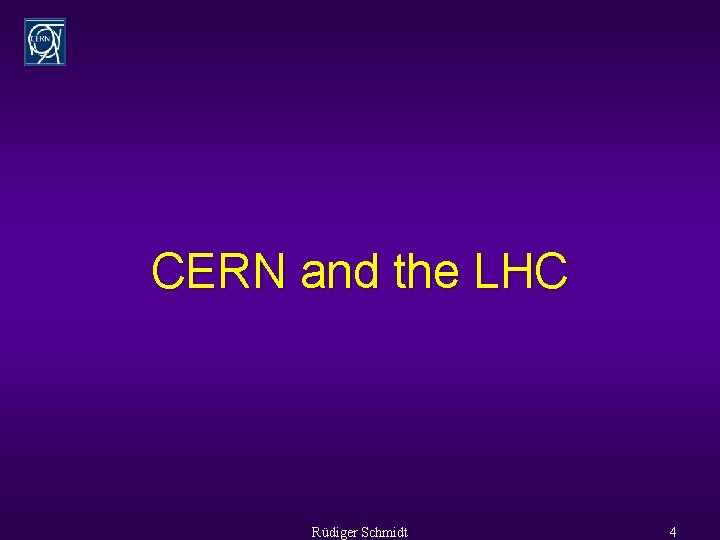
CERN and the LHC Rüdiger Schmidt 4
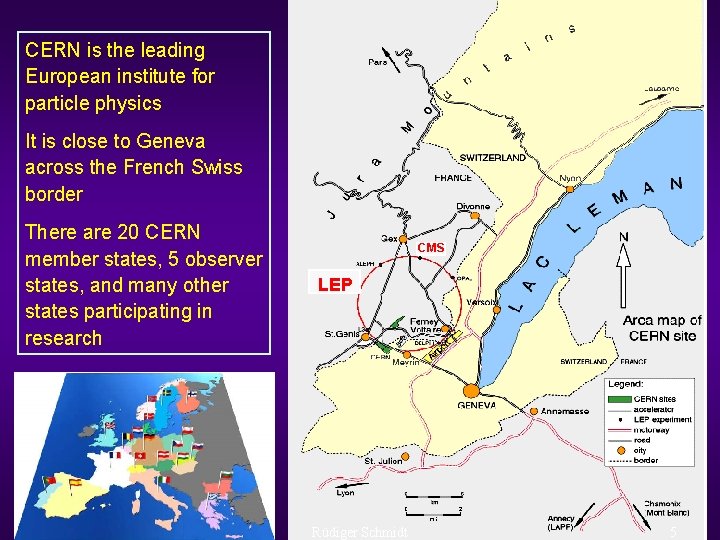
CERN is the leading European institute for particle physics It is close to Geneva across the French Swiss border There are 20 CERN member states, 5 observer states, and many other states participating in research CMS LEP Rüdiger Schmidt 5
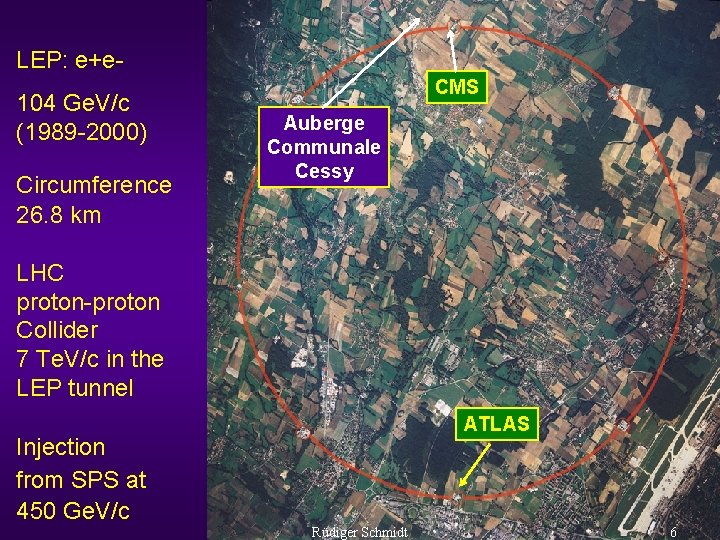
LEP: e+e 104 Ge. V/c (1989 -2000) Circumference 26. 8 km CMS Auberge Communale Cessy LHC proton-proton Collider 7 Te. V/c in the LEP tunnel Injection from SPS at 450 Ge. V/c ATLAS Rüdiger Schmidt 6
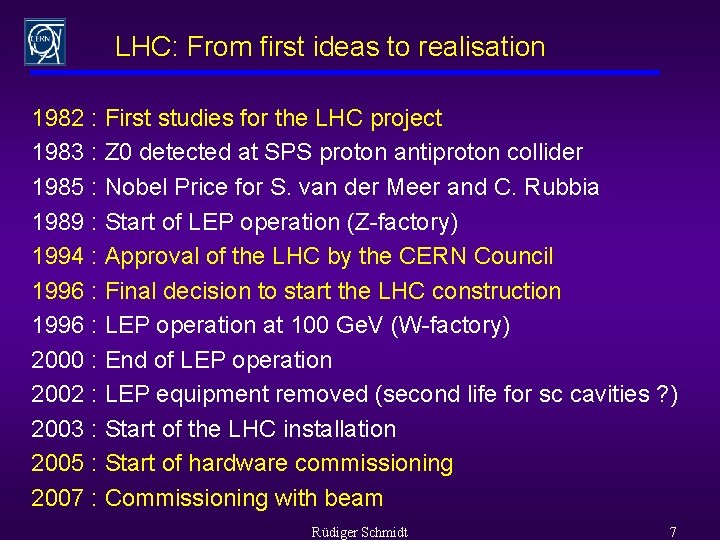
LHC: From first ideas to realisation 1982 : First studies for the LHC project 1983 : Z 0 detected at SPS proton antiproton collider 1985 : Nobel Price for S. van der Meer and C. Rubbia 1989 : Start of LEP operation (Z-factory) 1994 : Approval of the LHC by the CERN Council 1996 : Final decision to start the LHC construction 1996 : LEP operation at 100 Ge. V (W-factory) 2000 : End of LEP operation 2002 : LEP equipment removed (second life for sc cavities ? ) 2003 : Start of the LHC installation 2005 : Start of hardware commissioning 2007 : Commissioning with beam Rüdiger Schmidt 7
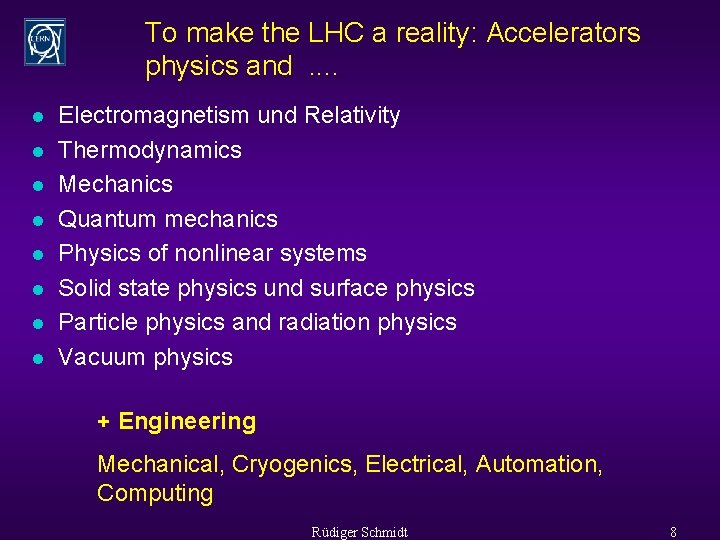
To make the LHC a reality: Accelerators physics and. . l l l l Electromagnetism und Relativity Thermodynamics Mechanics Quantum mechanics Physics of nonlinear systems Solid state physics und surface physics Particle physics and radiation physics Vacuum physics + Engineering Mechanical, Cryogenics, Electrical, Automation, Computing Rüdiger Schmidt 8
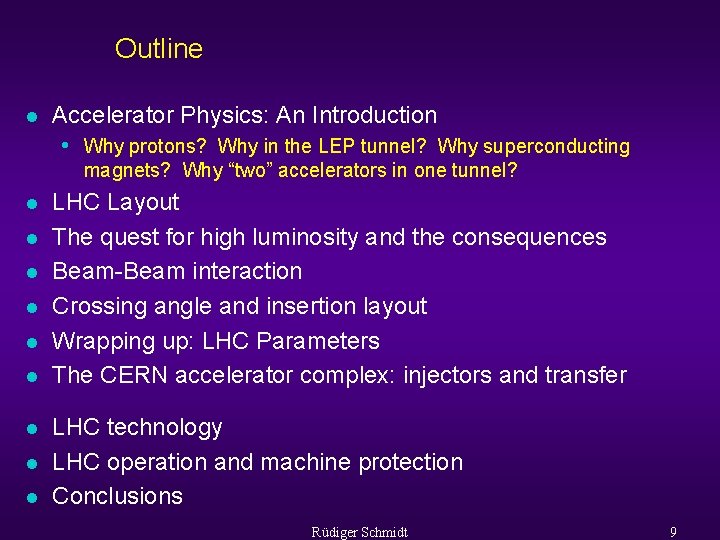
Outline l Accelerator Physics: An Introduction • Why protons? Why in the LEP tunnel? Why superconducting magnets? Why “two” accelerators in one tunnel? l l l l l LHC Layout The quest for high luminosity and the consequences Beam-Beam interaction Crossing angle and insertion layout Wrapping up: LHC Parameters The CERN accelerator complex: injectors and transfer LHC technology LHC operation and machine protection Conclusions Rüdiger Schmidt 9
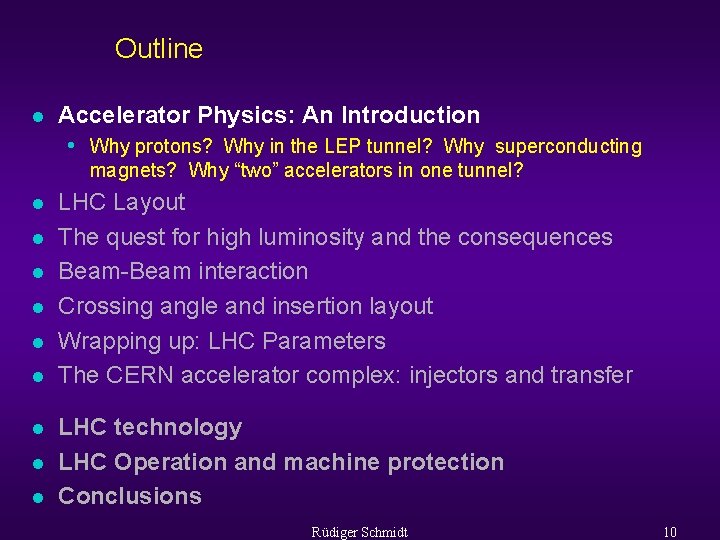
Outline l Accelerator Physics: An Introduction • Why protons? Why in the LEP tunnel? Why superconducting magnets? Why “two” accelerators in one tunnel? l l l l l LHC Layout The quest for high luminosity and the consequences Beam-Beam interaction Crossing angle and insertion layout Wrapping up: LHC Parameters The CERN accelerator complex: injectors and transfer LHC technology LHC Operation and machine protection Conclusions Rüdiger Schmidt 10
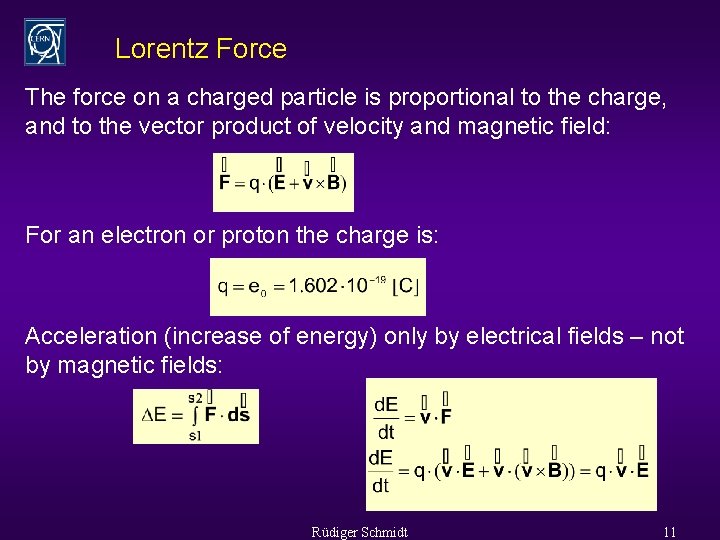
Lorentz Force The force on a charged particle is proportional to the charge, and to the vector product of velocity and magnetic field: For an electron or proton the charge is: Acceleration (increase of energy) only by electrical fields – not by magnetic fields: Rüdiger Schmidt 11
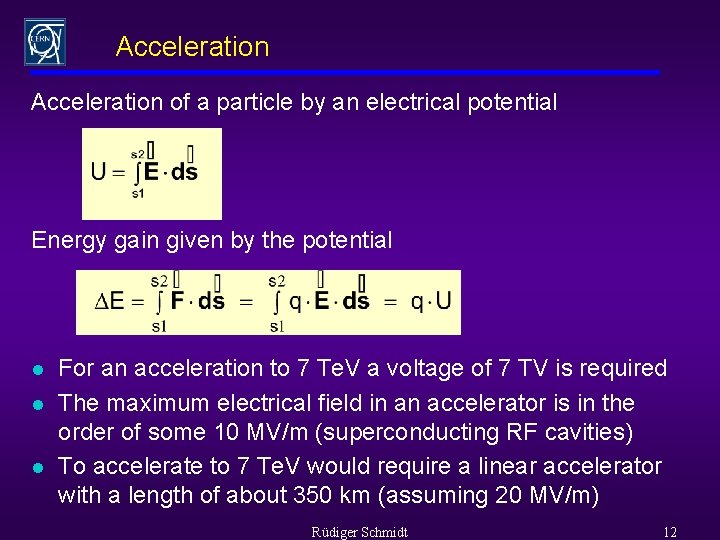
Acceleration of a particle by an electrical potential Energy gain given by the potential l For an acceleration to 7 Te. V a voltage of 7 TV is required The maximum electrical field in an accelerator is in the order of some 10 MV/m (superconducting RF cavities) To accelerate to 7 Te. V would require a linear accelerator with a length of about 350 km (assuming 20 MV/m) Rüdiger Schmidt 12
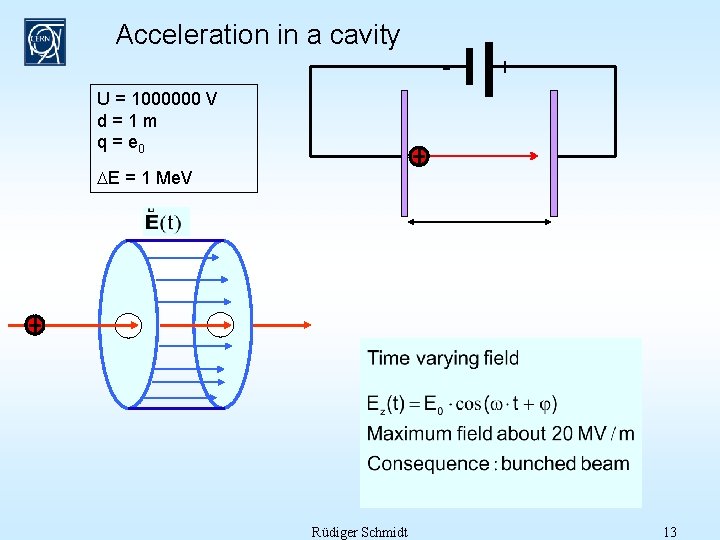
Acceleration in a cavity - + U = 1000000 V d=1 m q = e 0 E = 1 Me. V Rüdiger Schmidt 13
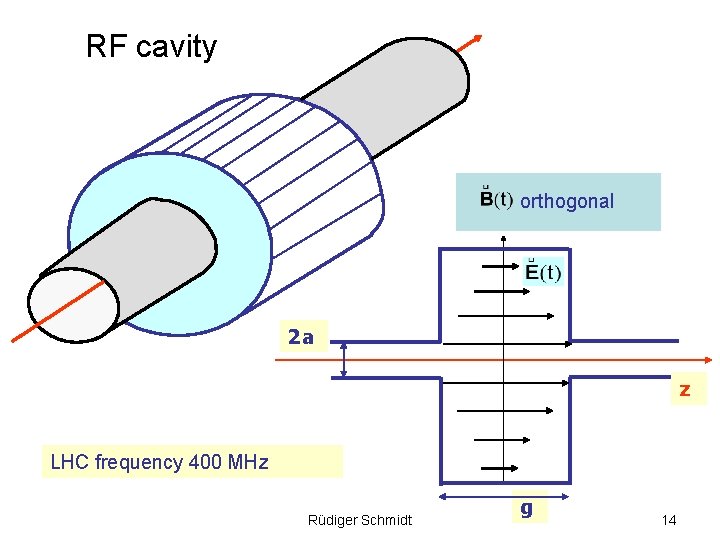
RF cavity orthogonal 2 a z LHC frequency 400 MHz Rüdiger Schmidt g 14
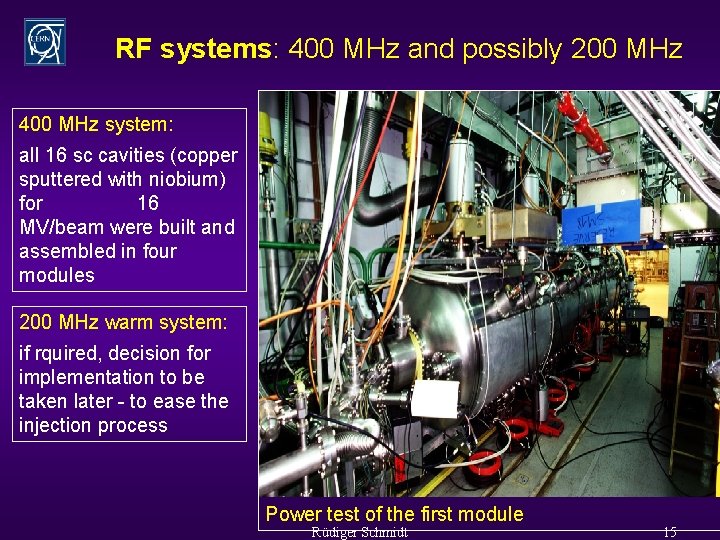
RF systems: 400 MHz and possibly 200 MHz 400 MHz system: all 16 sc cavities (copper sputtered with niobium) for 16 MV/beam were built and assembled in four modules 200 MHz warm system: if rquired, decision for implementation to be taken later - to ease the injection process Power test of the first module Rüdiger Schmidt 15
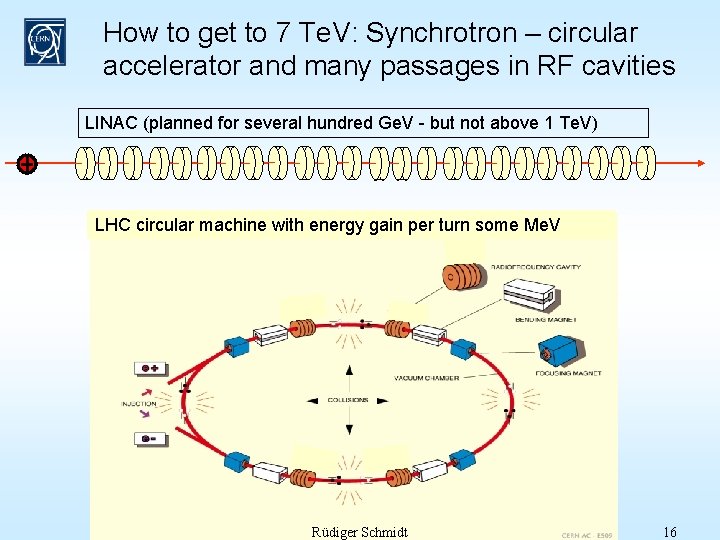
How to get to 7 Te. V: Synchrotron – circular accelerator and many passages in RF cavities LINAC (planned for several hundred Ge. V - but not above 1 Te. V) LHC circular machine with energy gain per turn some Me. V Rüdiger Schmidt 16
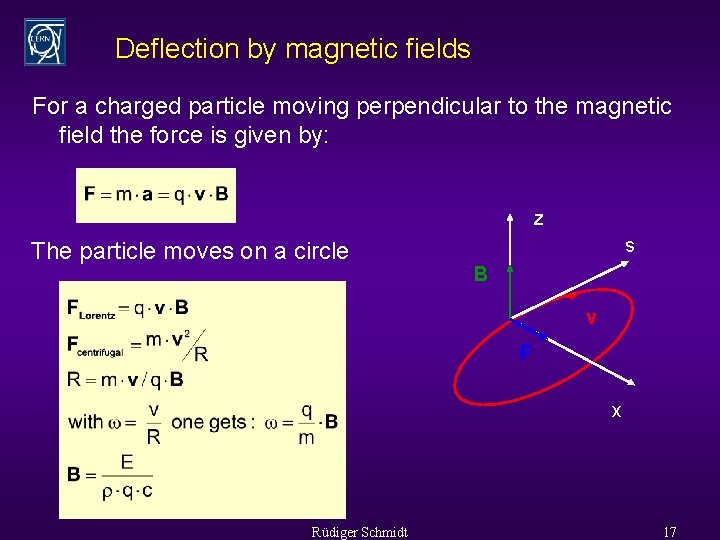
Deflection by magnetic fields For a charged particle moving perpendicular to the magnetic field the force is given by: z The particle moves on a circle s B v F x Rüdiger Schmidt 17
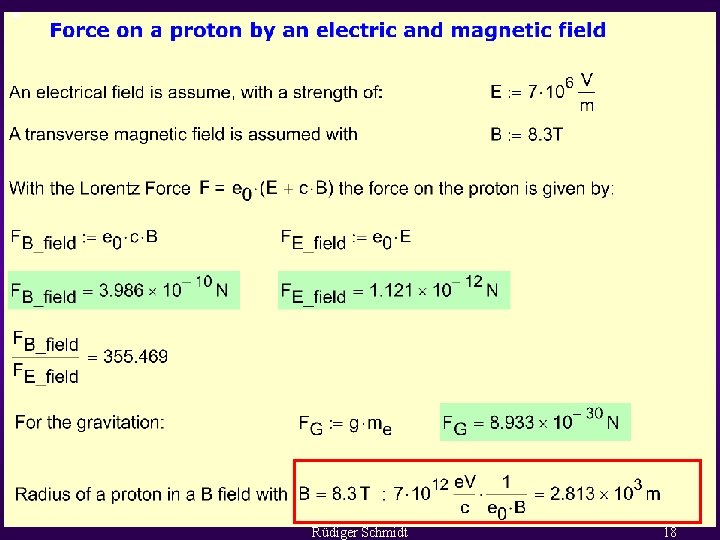
Deflection by magnetic fields Rüdiger Schmidt 18
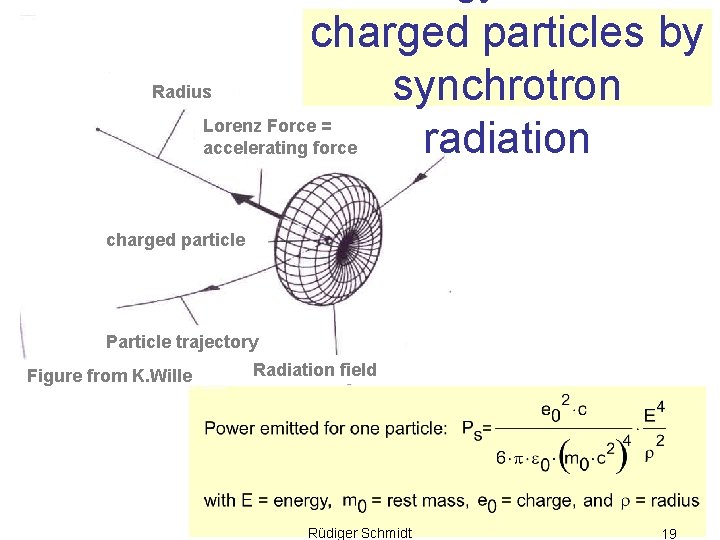
charged particles by Radius synchrotron Lorenz Force = radiation accelerating force charged particle Particle trajectory Figure from K. Wille Radiation field Rüdiger Schmidt 19
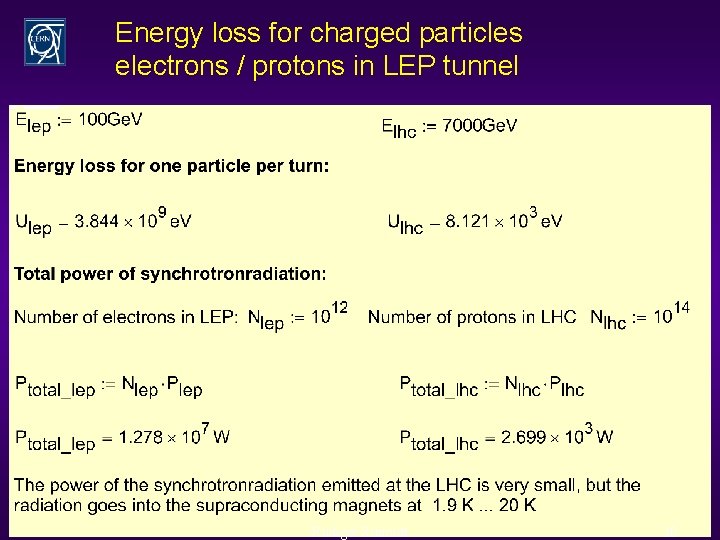
Energy loss for charged particles electrons / protons in LEP tunnel Rüdiger Schmidt 20
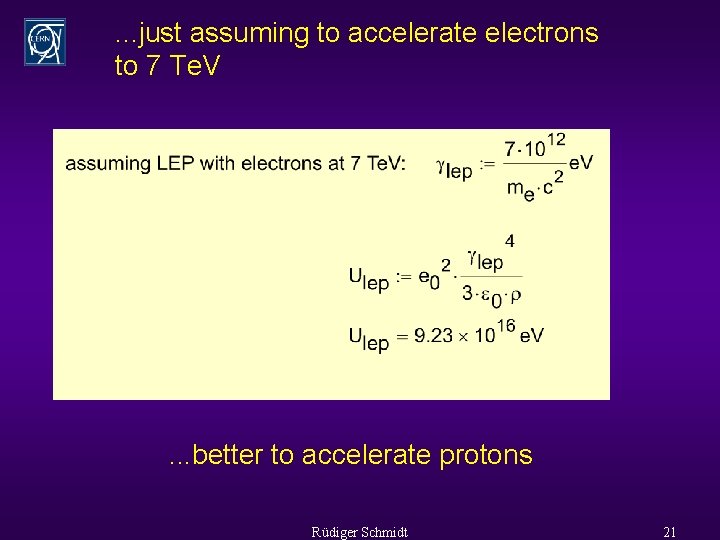
. . . just assuming to accelerate electrons to 7 Te. V . . . better to accelerate protons Rüdiger Schmidt 21
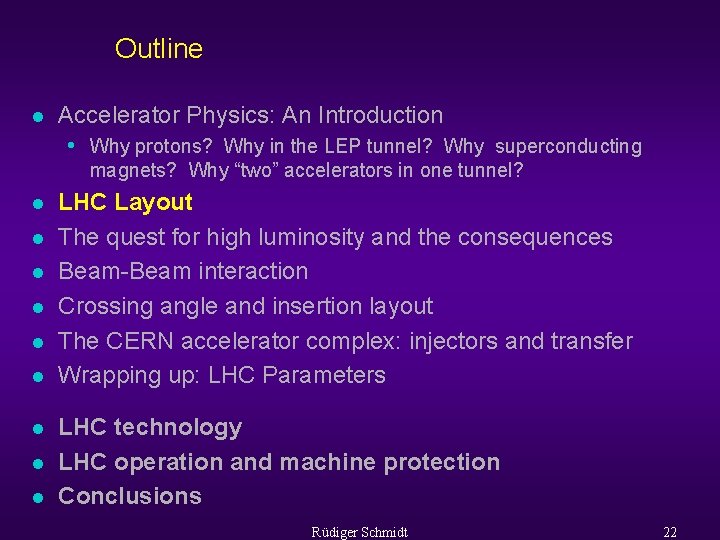
Outline l Accelerator Physics: An Introduction • Why protons? Why in the LEP tunnel? Why superconducting magnets? Why “two” accelerators in one tunnel? l l l l l LHC Layout The quest for high luminosity and the consequences Beam-Beam interaction Crossing angle and insertion layout The CERN accelerator complex: injectors and transfer Wrapping up: LHC Parameters LHC technology LHC operation and machine protection Conclusions Rüdiger Schmidt 22
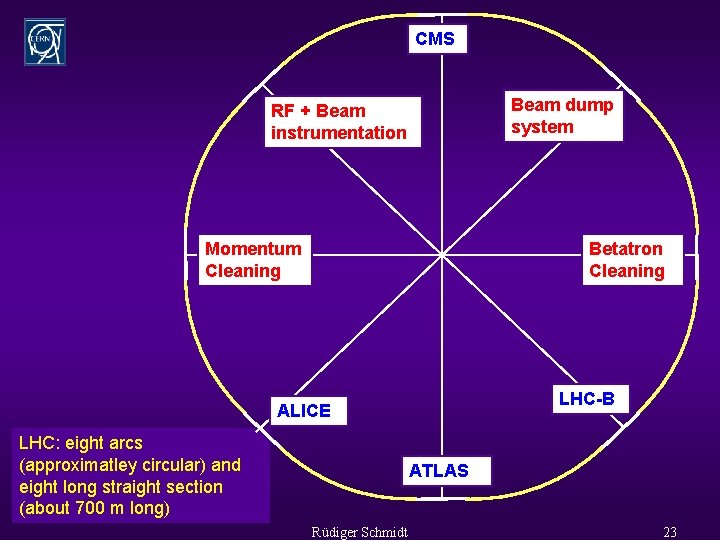
CMS Beam dump system RF + Beam instrumentation Momentum Cleaning Betatron Cleaning LHC-B ALICE LHC: eight arcs (approximatley circular) and eight long straight section (about 700 m long) ATLAS Rüdiger Schmidt 23
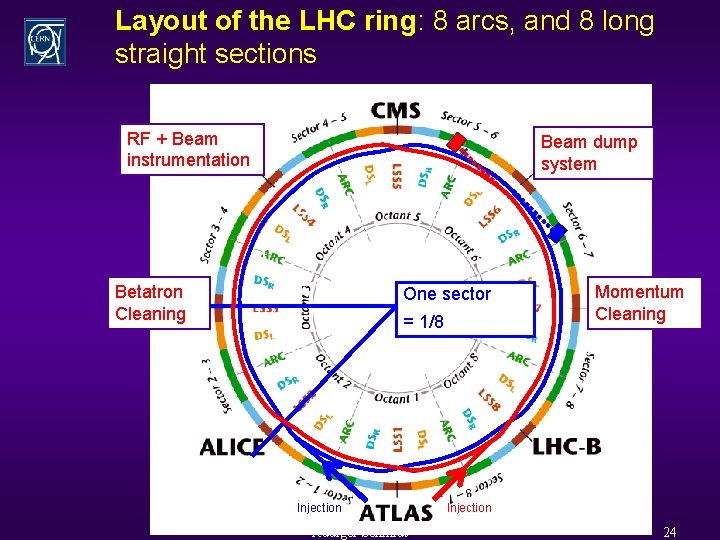
Layout of the LHC ring: 8 arcs, and 8 long straight sections RF + Beam instrumentation Beam dump system Betatron Cleaning One sector = 1/8 Injection Rüdiger Schmidt Momentum Cleaning Injection 24
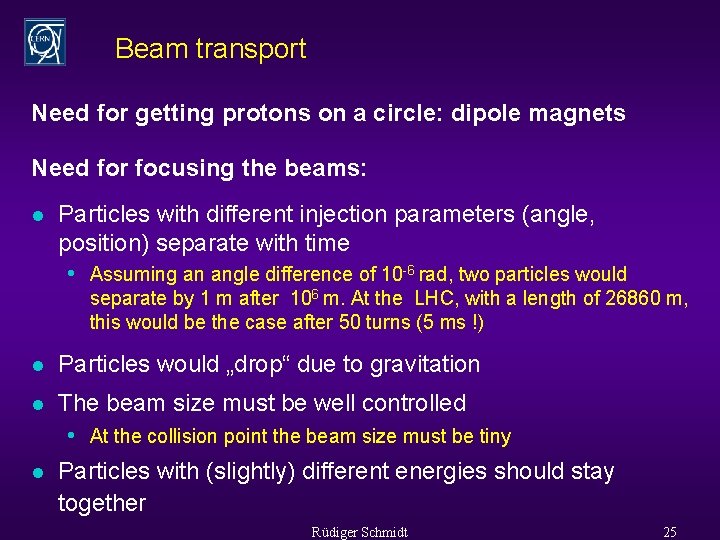
Beam transport Need for getting protons on a circle: dipole magnets Need for focusing the beams: l Particles with different injection parameters (angle, position) separate with time • Assuming an angle difference of 10 -6 rad, two particles would separate by 1 m after 106 m. At the LHC, with a length of 26860 m, this would be the case after 50 turns (5 ms !) l Particles would „drop“ due to gravitation l The beam size must be well controlled • At the collision point the beam size must be tiny l Particles with (slightly) different energies should stay together Rüdiger Schmidt 25
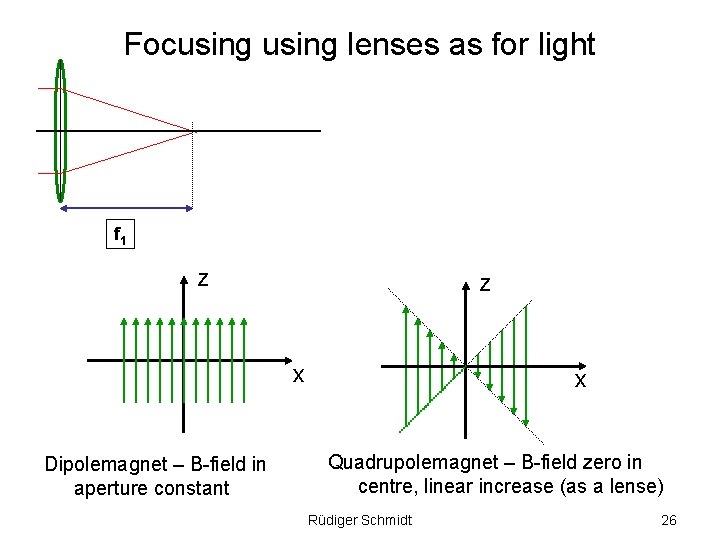
Focusing lenses as for light f 1 z z x Dipolemagnet – B-field in aperture constant x Quadrupolemagnet – B-field zero in centre, linear increase (as a lense) Rüdiger Schmidt 26
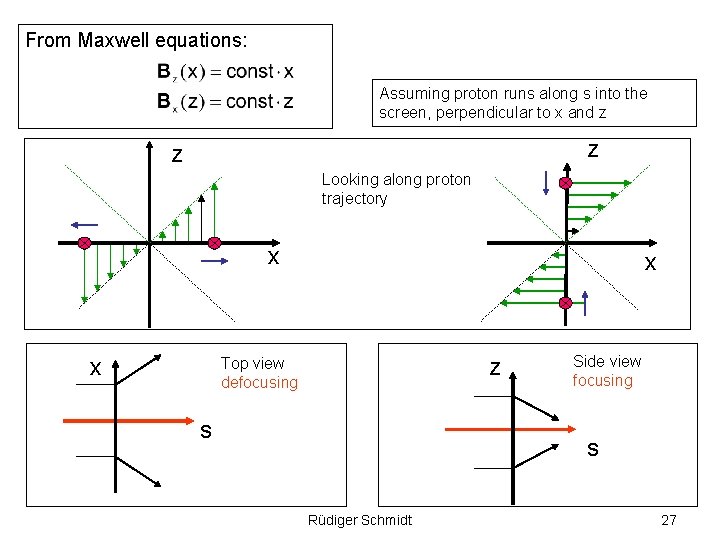
From Maxwell equations: Assuming proton runs along s into the screen, perpendicular to x and z z z Looking along proton trajectory x x x z Top view defocusing s Side view focusing s Rüdiger Schmidt 27
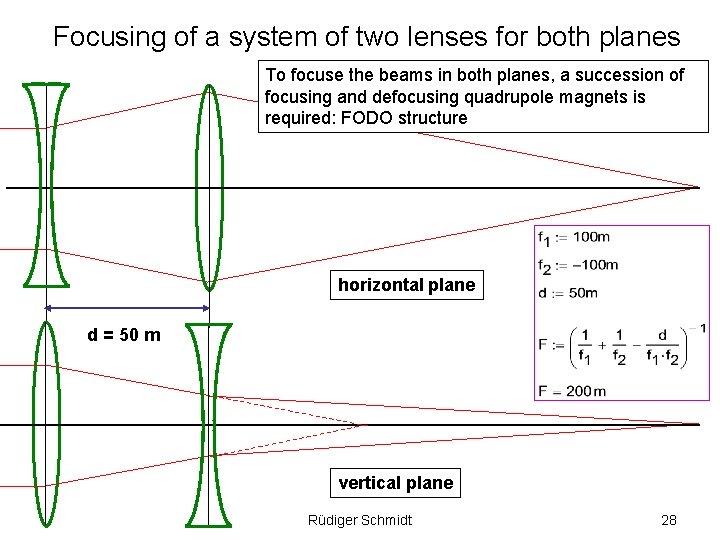
Focusing of a system of two lenses for both planes To focuse the beams in both planes, a succession of focusing and defocusing quadrupole magnets is required: FODO structure horizontal plane d = 50 m vertical plane Rüdiger Schmidt 28
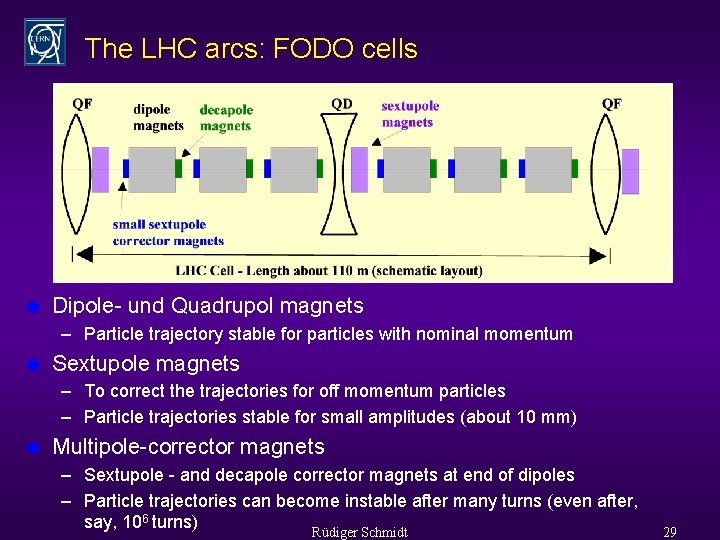
The LHC arcs: FODO cells u Dipole- und Quadrupol magnets – Particle trajectory stable for particles with nominal momentum u Sextupole magnets – To correct the trajectories for off momentum particles – Particle trajectories stable for small amplitudes (about 10 mm) u Multipole-corrector magnets – Sextupole - and decapole corrector magnets at end of dipoles – Particle trajectories can become instable after many turns (even after, say, 106 turns) Rüdiger Schmidt 29
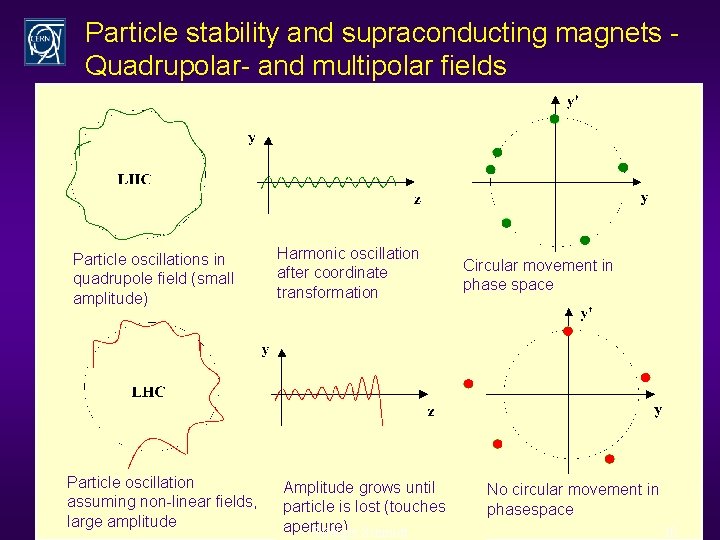
Particle stability and supraconducting magnets Quadrupolar- and multipolar fields Particle oscillations in quadrupole field (small amplitude) Particle oscillation assuming non-linear fields, large amplitude Harmonic oscillation after coordinate transformation Amplitude grows until particle is lost (touches aperture) Rüdiger Schmidt Circular movement in phase space No circular movement in phasespace 30
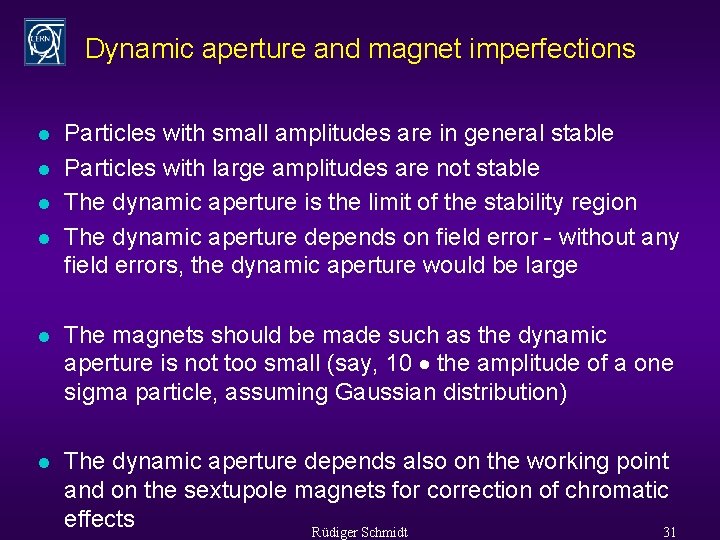
Dynamic aperture and magnet imperfections l l Particles with small amplitudes are in general stable Particles with large amplitudes are not stable The dynamic aperture is the limit of the stability region The dynamic aperture depends on field error - without any field errors, the dynamic aperture would be large l The magnets should be made such as the dynamic aperture is not too small (say, 10 the amplitude of a one sigma particle, assuming Gaussian distribution) l The dynamic aperture depends also on the working point and on the sextupole magnets for correction of chromatic effects Rüdiger Schmidt 31
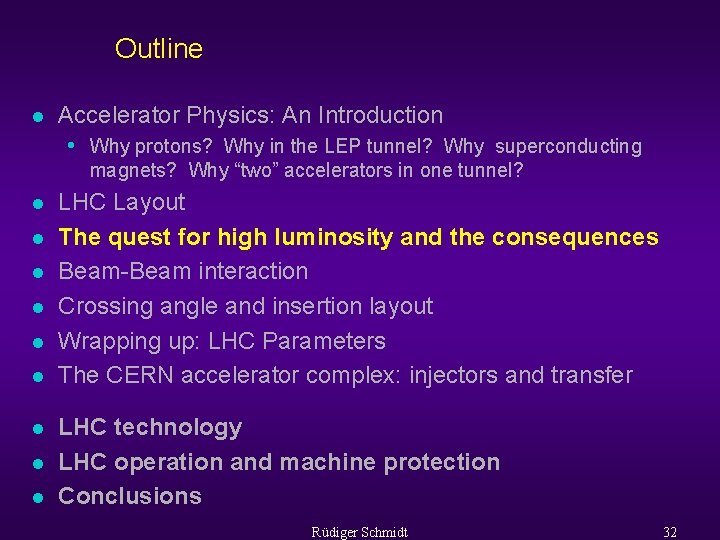
Outline l Accelerator Physics: An Introduction • Why protons? Why in the LEP tunnel? Why superconducting magnets? Why “two” accelerators in one tunnel? l l l l l LHC Layout The quest for high luminosity and the consequences Beam-Beam interaction Crossing angle and insertion layout Wrapping up: LHC Parameters The CERN accelerator complex: injectors and transfer LHC technology LHC operation and machine protection Conclusions Rüdiger Schmidt 32
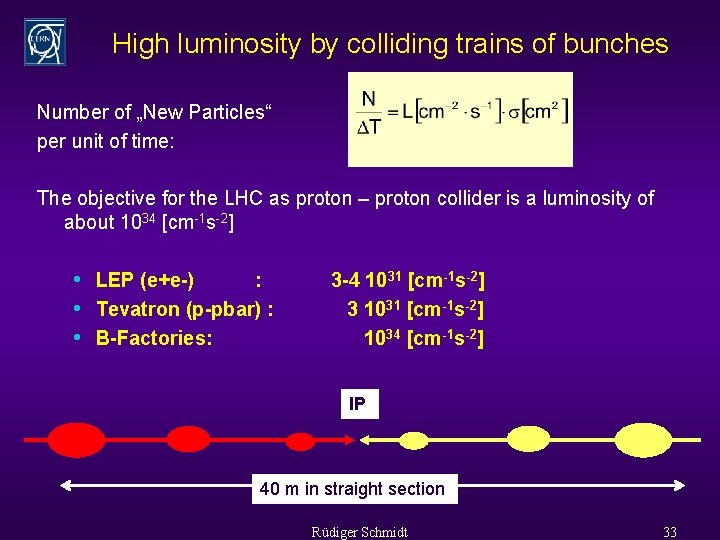
High luminosity by colliding trains of bunches Number of „New Particles“ per unit of time: The objective for the LHC as proton – proton collider is a luminosity of about 1034 [cm-1 s-2] • LEP (e+e-) : • Tevatron (p-pbar) : • B-Factories: 3 -4 1031 [cm-1 s-2] 3 1031 [cm-1 s-2] 1034 [cm-1 s-2] IP 40 m in straight section Rüdiger Schmidt 33
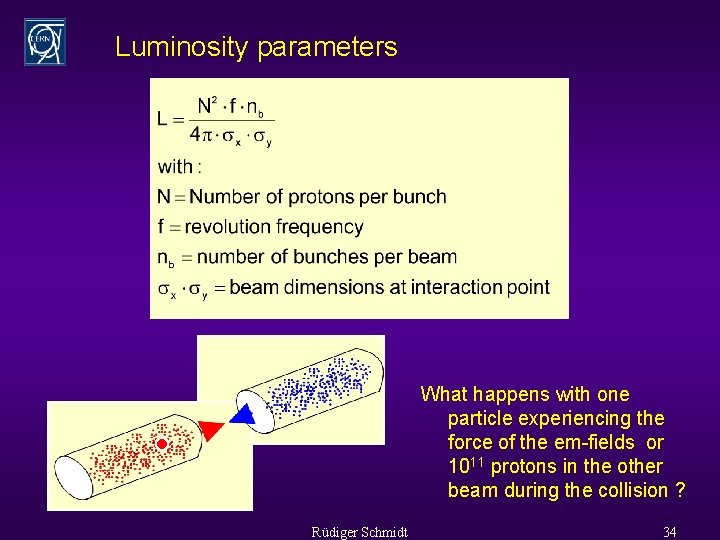
Luminosity parameters What happens with one particle experiencing the force of the em-fields or 1011 protons in the other beam during the collision ? Rüdiger Schmidt 34
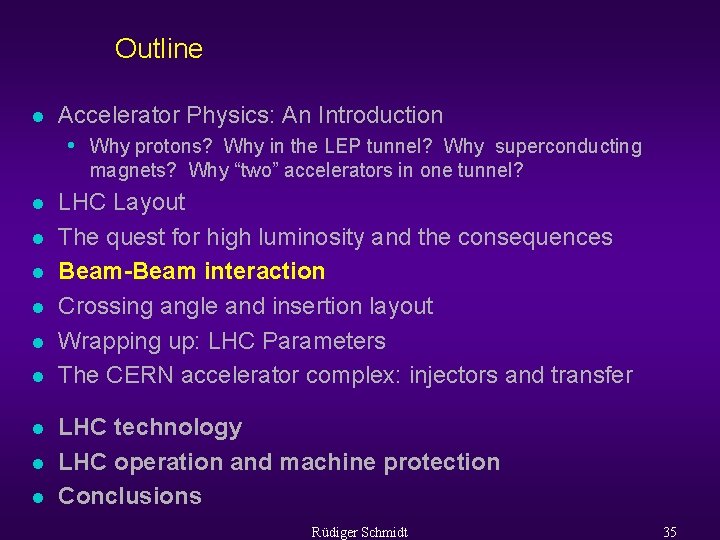
Outline l Accelerator Physics: An Introduction • Why protons? Why in the LEP tunnel? Why superconducting magnets? Why “two” accelerators in one tunnel? l l l l l LHC Layout The quest for high luminosity and the consequences Beam-Beam interaction Crossing angle and insertion layout Wrapping up: LHC Parameters The CERN accelerator complex: injectors and transfer LHC technology LHC operation and machine protection Conclusions Rüdiger Schmidt 35
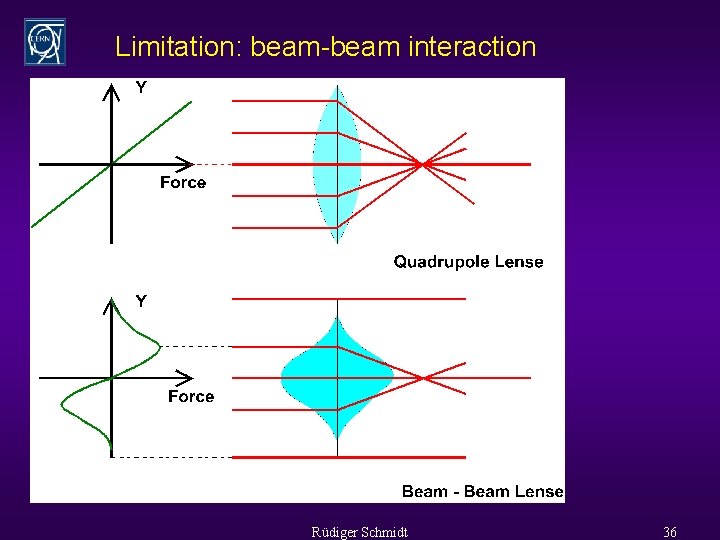
Limitation: beam-beam interaction Rüdiger Schmidt 36
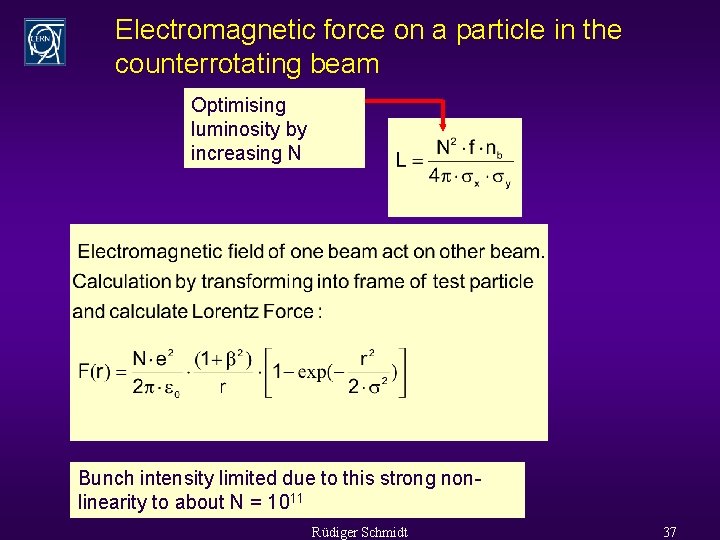
Electromagnetic force on a particle in the counterrotating beam Optimising luminosity by increasing N Bunch intensity limited due to this strong nonlinearity to about N = 1011 Rüdiger Schmidt 37
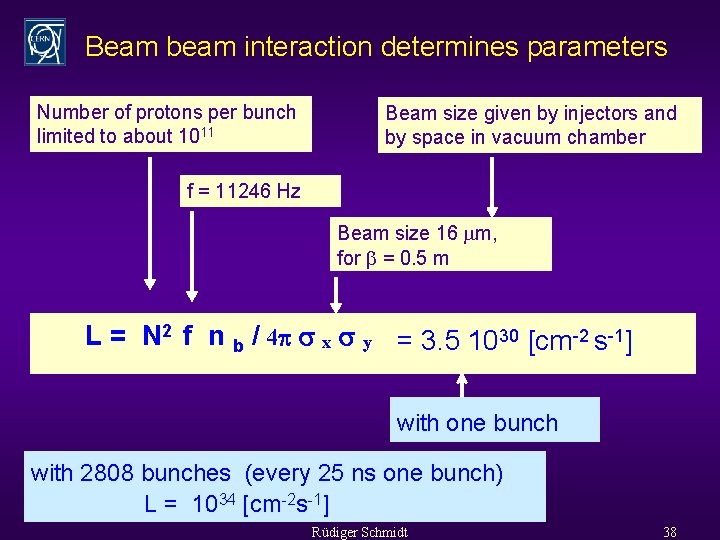
Beam beam interaction determines parameters Number of protons per bunch limited to about 1011 Beam size given by injectors and by space in vacuum chamber f = 11246 Hz Beam size 16 m, for = 0. 5 m L = N 2 f n b / 4 p s x s y = 3. 5 1030 [cm-2 s-1] with one bunch with 2808 bunches (every 25 ns one bunch) L = 1034 [cm-2 s-1] Rüdiger Schmidt 38
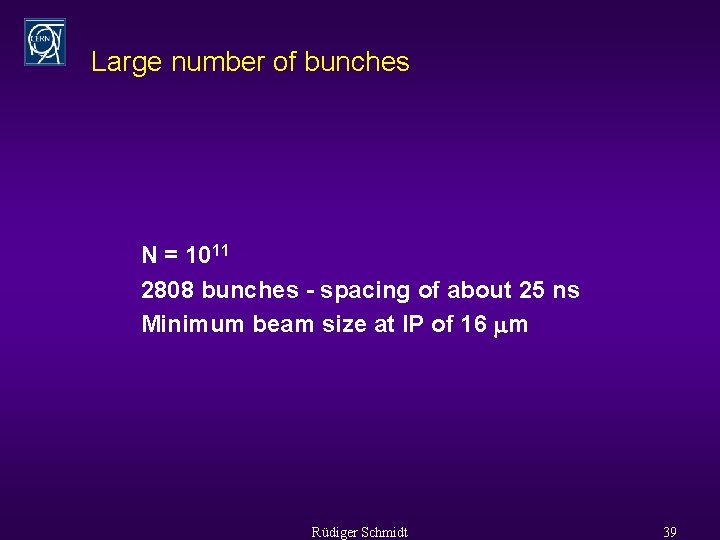
Large number of bunches N = 1011 2808 bunches - spacing of about 25 ns Minimum beam size at IP of 16 m Rüdiger Schmidt 39
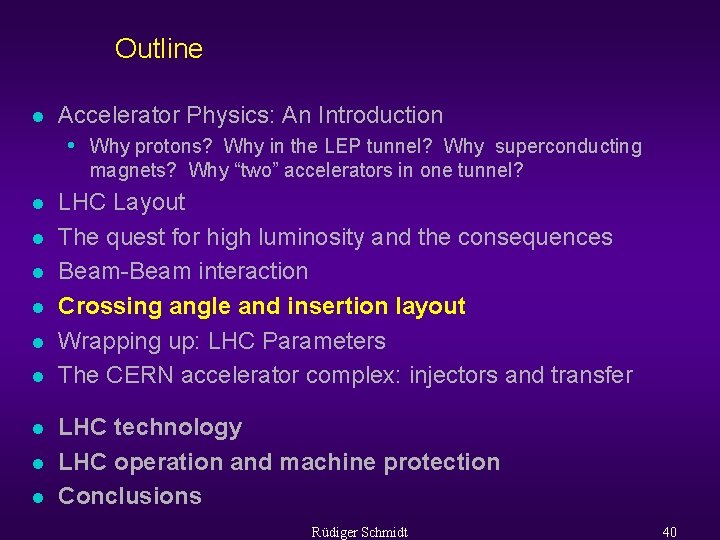
Outline l Accelerator Physics: An Introduction • Why protons? Why in the LEP tunnel? Why superconducting magnets? Why “two” accelerators in one tunnel? l l l l l LHC Layout The quest for high luminosity and the consequences Beam-Beam interaction Crossing angle and insertion layout Wrapping up: LHC Parameters The CERN accelerator complex: injectors and transfer LHC technology LHC operation and machine protection Conclusions Rüdiger Schmidt 40
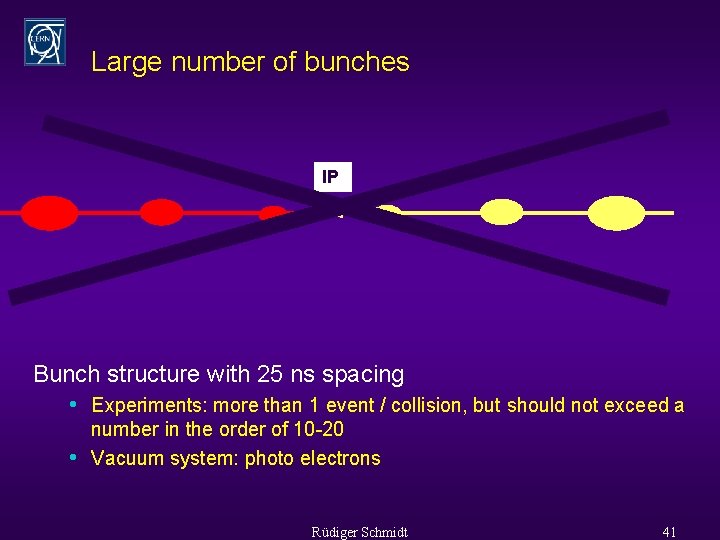
Large number of bunches IP Bunch structure with 25 ns spacing • Experiments: more than 1 event / collision, but should not exceed a • number in the order of 10 -20 Vacuum system: photo electrons Rüdiger Schmidt 41
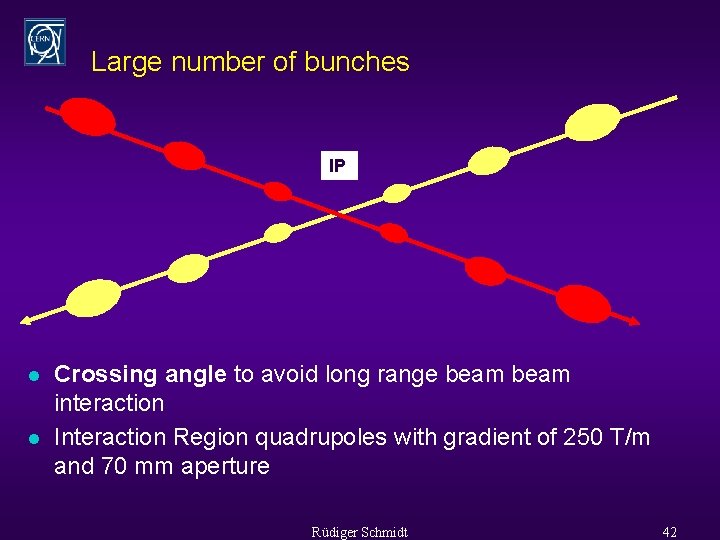
Large number of bunches IP l l Crossing angle to avoid long range beam interaction Interaction Region quadrupoles with gradient of 250 T/m and 70 mm aperture Rüdiger Schmidt 42
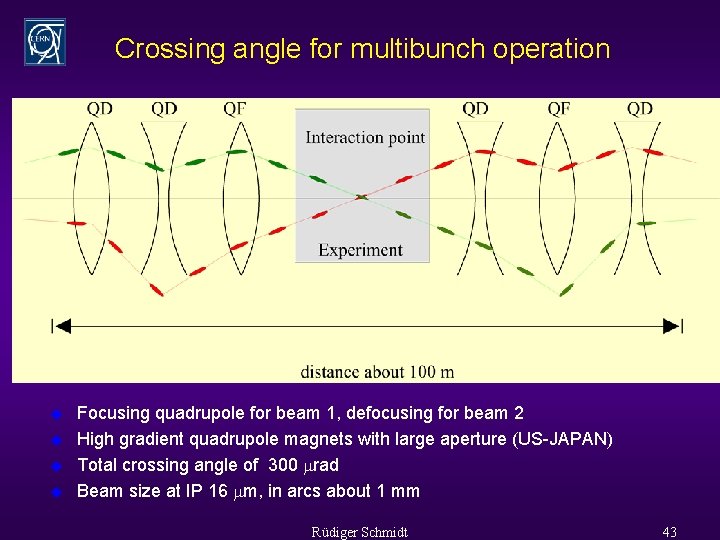
Crossing angle for multibunch operation u u Focusing quadrupole for beam 1, defocusing for beam 2 High gradient quadrupole magnets with large aperture (US-JAPAN) Total crossing angle of 300 rad Beam size at IP 16 m, in arcs about 1 mm Rüdiger Schmidt 43
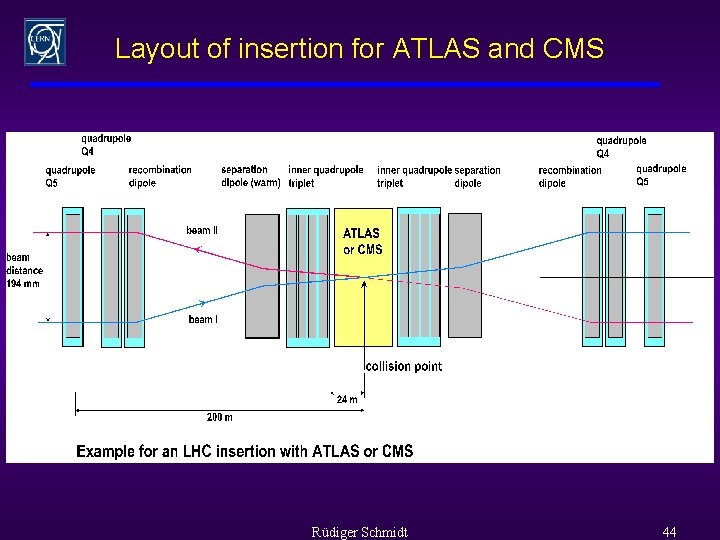
Layout of insertion for ATLAS and CMS Rüdiger Schmidt 44
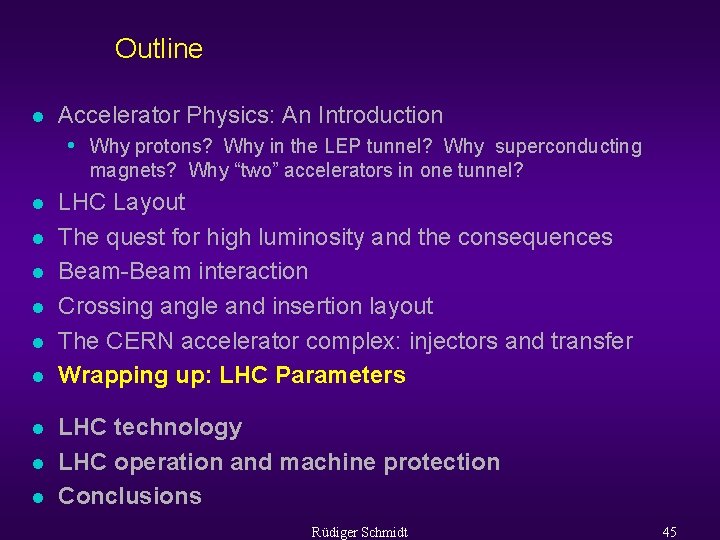
Outline l Accelerator Physics: An Introduction • Why protons? Why in the LEP tunnel? Why superconducting magnets? Why “two” accelerators in one tunnel? l l l l l LHC Layout The quest for high luminosity and the consequences Beam-Beam interaction Crossing angle and insertion layout The CERN accelerator complex: injectors and transfer Wrapping up: LHC Parameters LHC technology LHC operation and machine protection Conclusions Rüdiger Schmidt 45
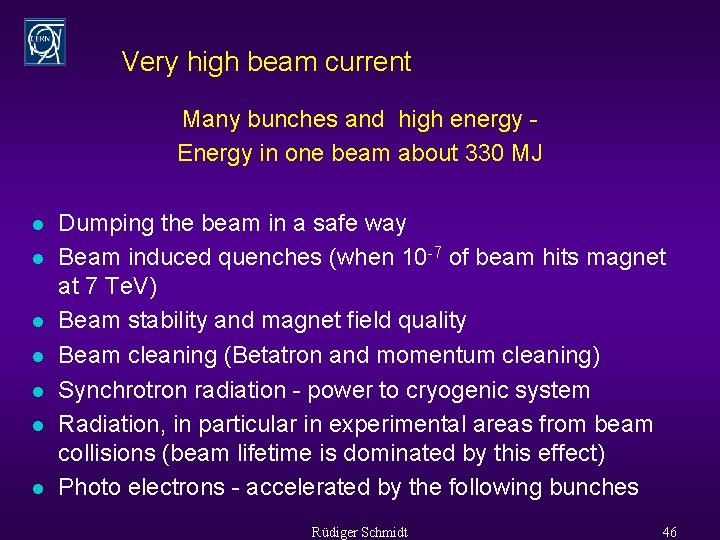
Very high beam current Many bunches and high energy Energy in one beam about 330 MJ l l l l Dumping the beam in a safe way Beam induced quenches (when 10 -7 of beam hits magnet at 7 Te. V) Beam stability and magnet field quality Beam cleaning (Betatron and momentum cleaning) Synchrotron radiation - power to cryogenic system Radiation, in particular in experimental areas from beam collisions (beam lifetime is dominated by this effect) Photo electrons - accelerated by the following bunches Rüdiger Schmidt 46
![Challenges Energy stored in the beam MJ One beam nominal intensity corresponds to an Challenges: Energy stored in the beam [MJ] One beam, nominal intensity (corresponds to an](https://slidetodoc.com/presentation_image_h2/3eba6217c3402601657c9dcc9384af2e/image-47.jpg)
Challenges: Energy stored in the beam [MJ] One beam, nominal intensity (corresponds to an energy that melts 500 kg of copper) x 200 x 10000 courtesy R. Assmann Momentum [Ge. V/c] Transverse energy density: Rüdiger even. Schmidt a factor of 1000 larger 47
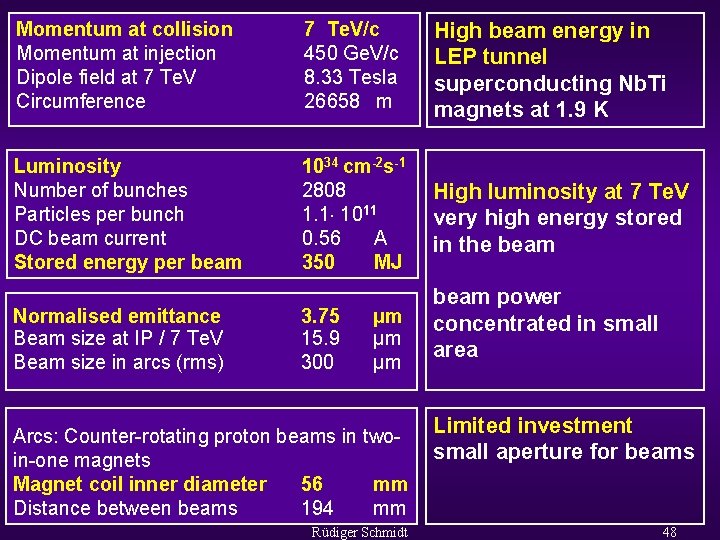
Momentum at collision Momentum at injection Dipole field at 7 Te. V Circumference 7 Te. V/c 450 Ge. V/c 8. 33 Tesla 26658 m High beam energy in LEP tunnel superconducting Nb. Ti magnets at 1. 9 K Luminosity Number of bunches Particles per bunch DC beam current Stored energy per beam 1034 cm-2 s-1 2808 1. 1 1011 0. 56 A 350 MJ High luminosity at 7 Te. V very high energy stored in the beam Normalised emittance Beam size at IP / 7 Te. V Beam size in arcs (rms) 3. 75 15. 9 300 µm µm µm Arcs: Counter-rotating proton beams in twoin-one magnets Magnet coil inner diameter 56 mm Distance between beams 194 mm Rüdiger Schmidt beam power concentrated in small area Limited investment small aperture for beams 48
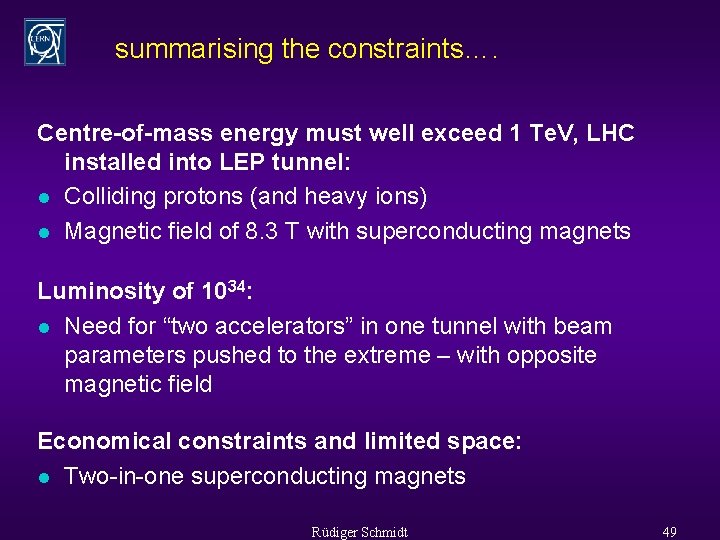
summarising the constraints…. Centre-of-mass energy must well exceed 1 Te. V, LHC installed into LEP tunnel: l Colliding protons (and heavy ions) l Magnetic field of 8. 3 T with superconducting magnets Luminosity of 1034: l Need for “two accelerators” in one tunnel with beam parameters pushed to the extreme – with opposite magnetic field Economical constraints and limited space: l Two-in-one superconducting magnets Rüdiger Schmidt 49
Cern future circular collider
Burkhard schmidt cern
Burkhard schmidt cern
Fcc collider
Fcc collider
Bnl
Muon collider
Particle collider
Unity sphere collider
Hadron collider
Hadron collider
International linear collider
Tevatron vs lhc
Lhc louisiana
Hl-lhc schedule
Forum lhc
Lhc logbook
Lhc morning meeting
Worldwide lhc computing grid
Lhc budget
Lhc schedule 2022
Lhc performance workshop
Lhc performance workshop
Lhc intranet
Hl-lhc schedule
Lhc performance workshop
Lhc filling scheme
Gant chart
"lhc"
Lhc
Lhc:8001692
Uuu dump
Lhc quench
Lhc beam dump
Forum lhc
"lhc"
"lhc"
Damien lafarge
Hadron
Lhc schedule
Lhc technical coordination
Hl-lhc schedule
Finsler geometry
Kme copper
Lhc plan
Hid cern
Hát kết hợp bộ gõ cơ thể
Ng-html
Bổ thể
Tỉ lệ cơ thể trẻ em