PLASMA ATOMIC LAYER ETCHING Ankur Agarwala and Mark
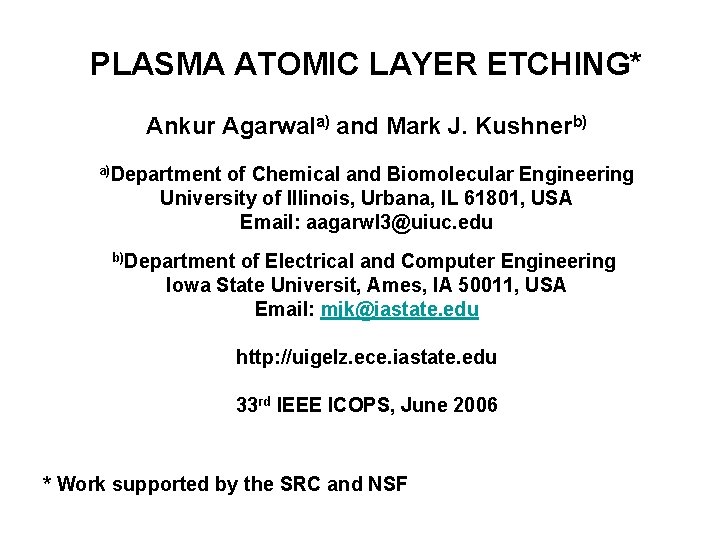
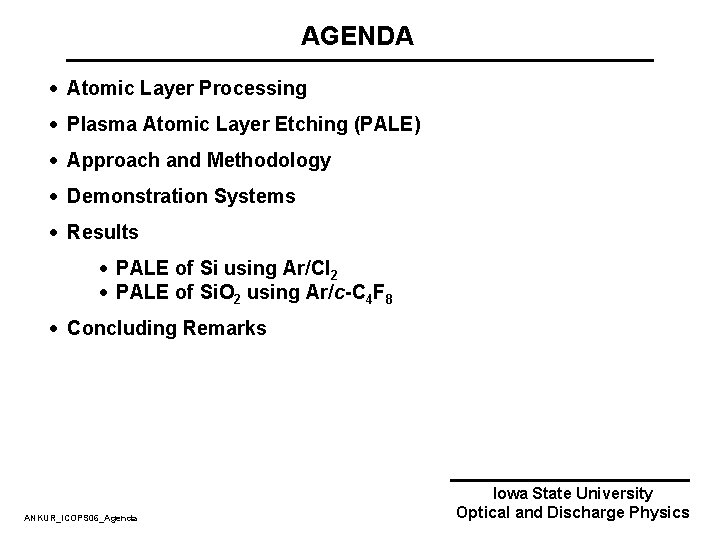
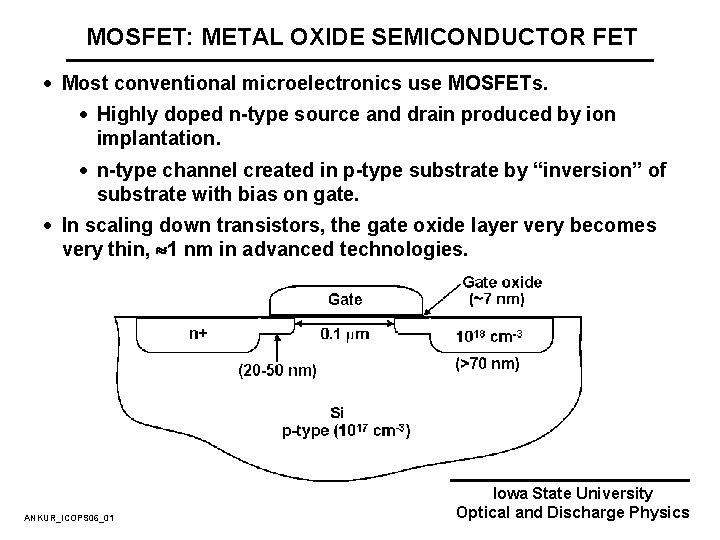
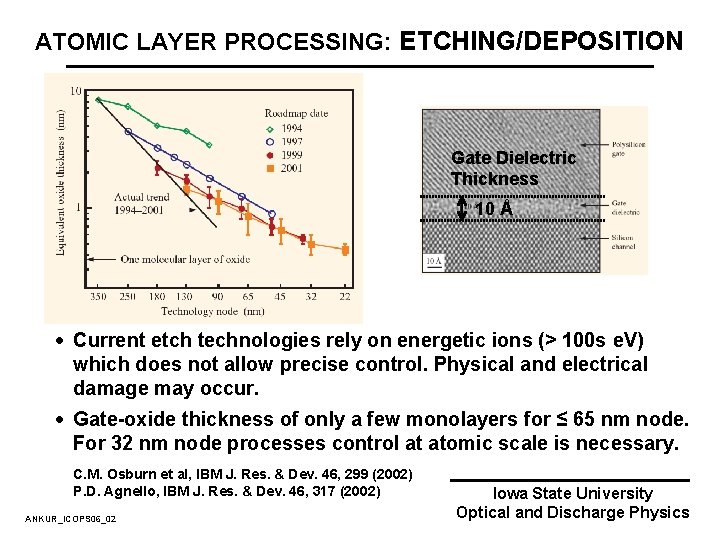
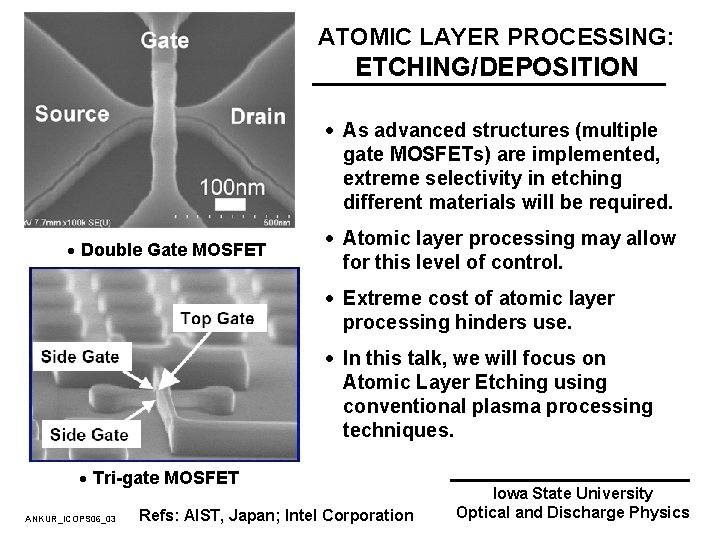
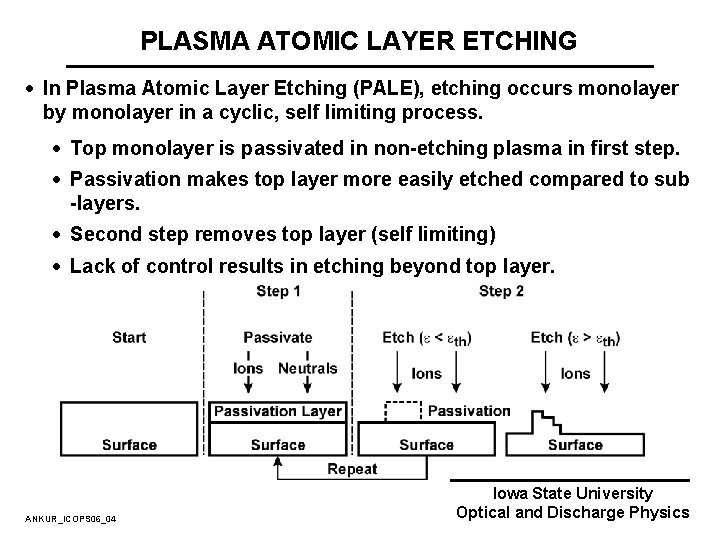
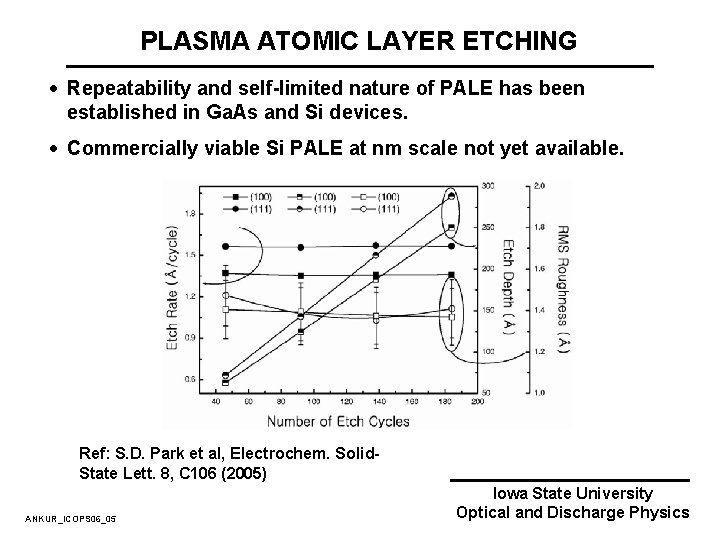
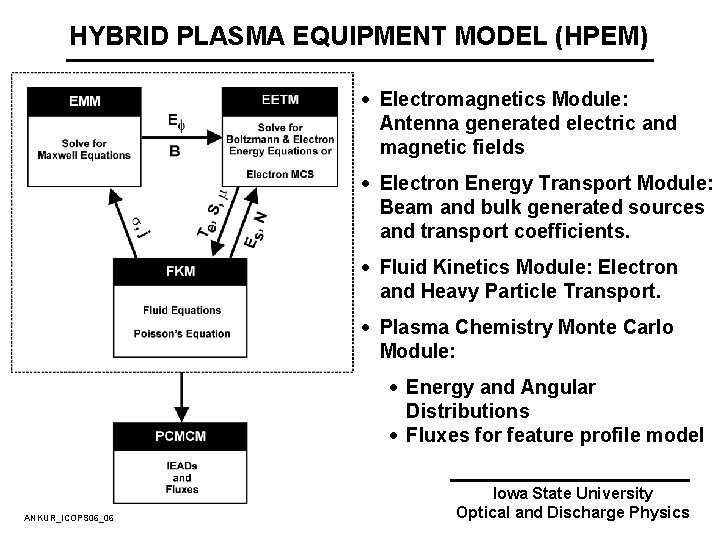
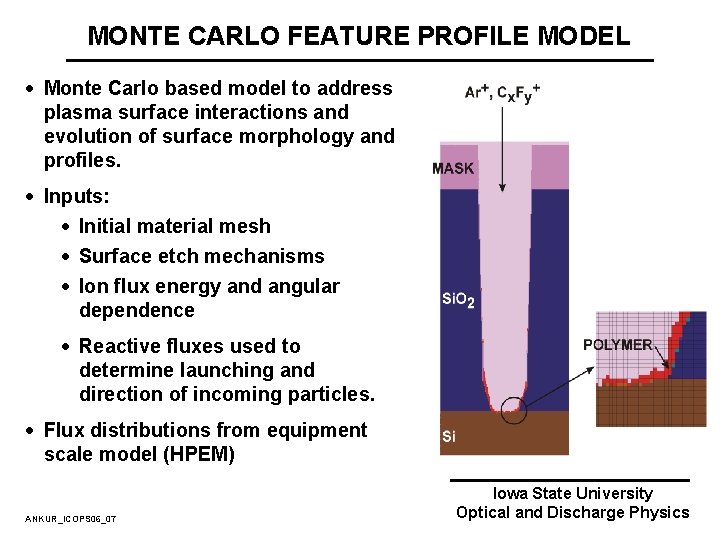
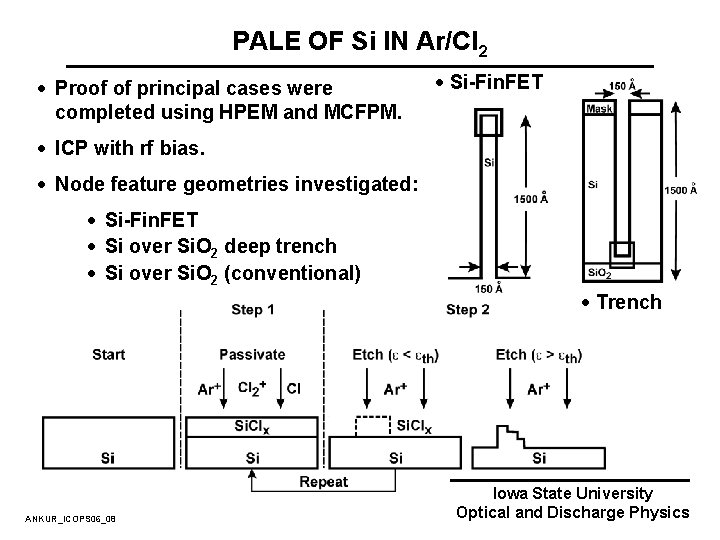
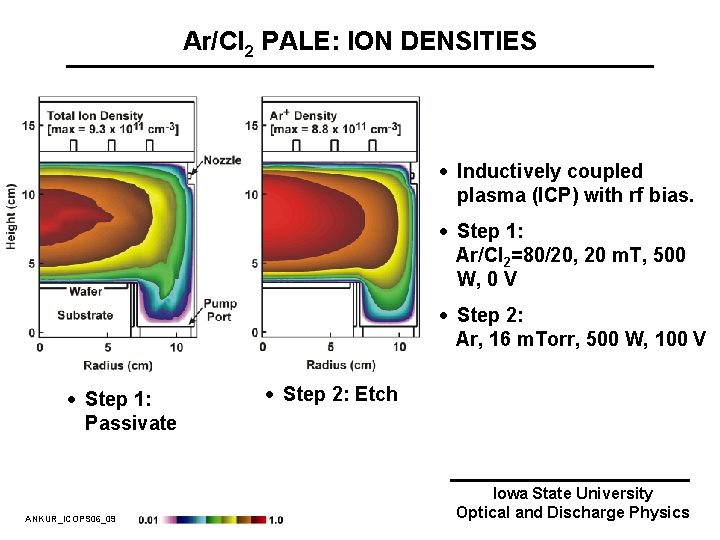
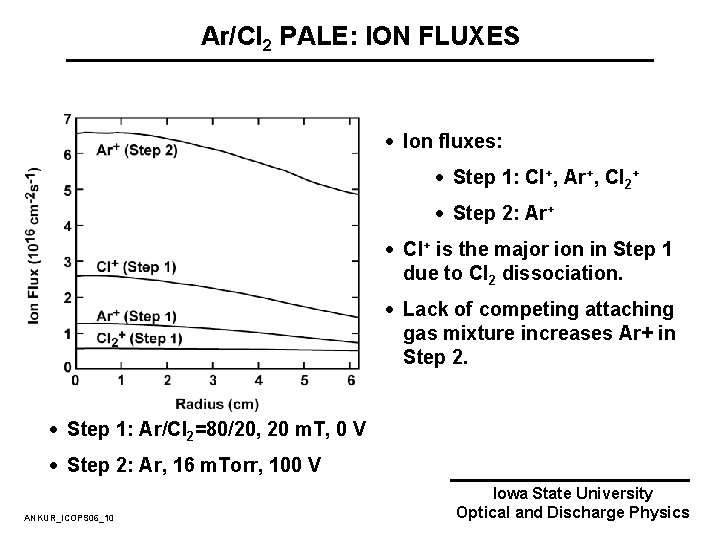
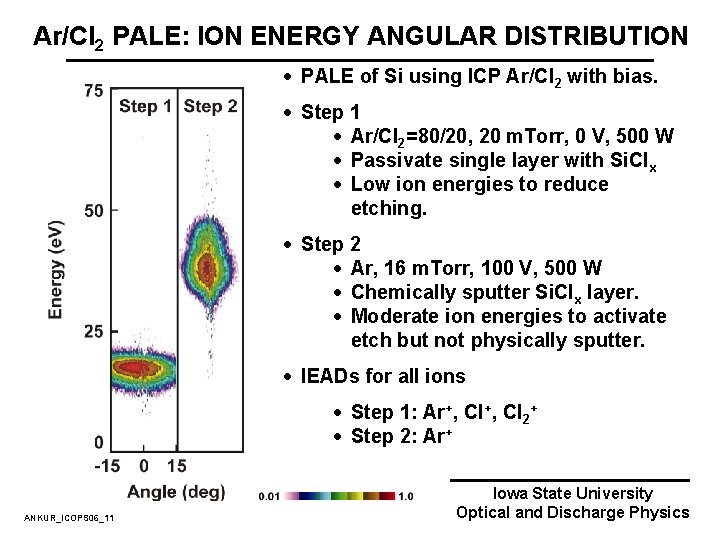
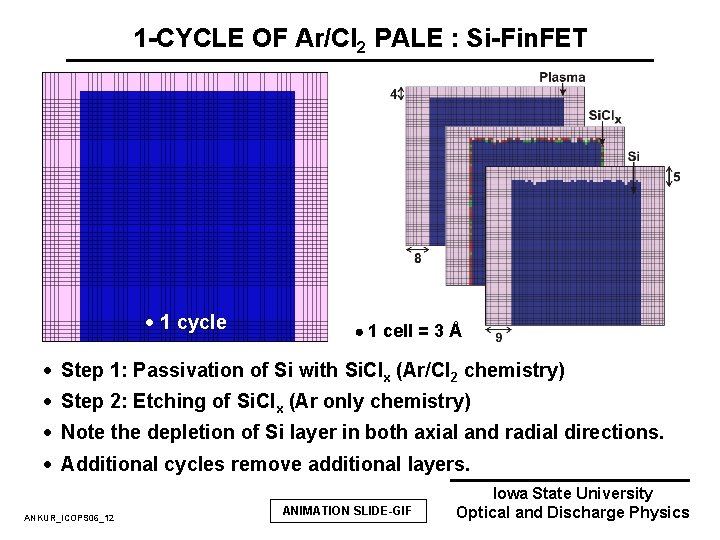
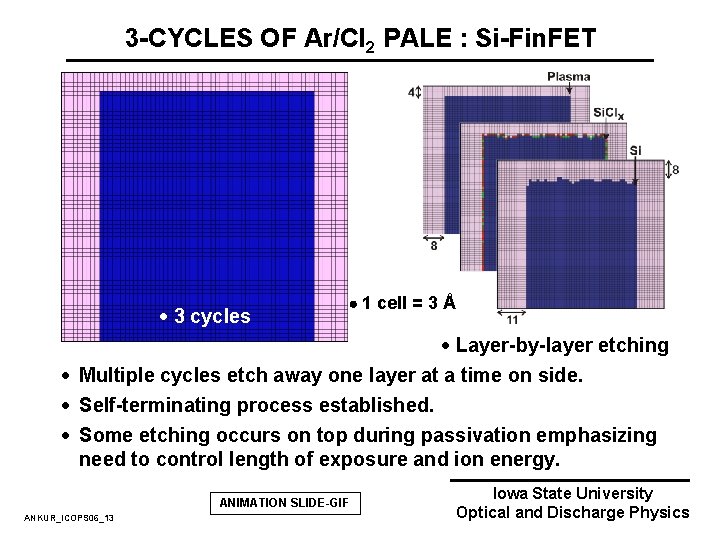
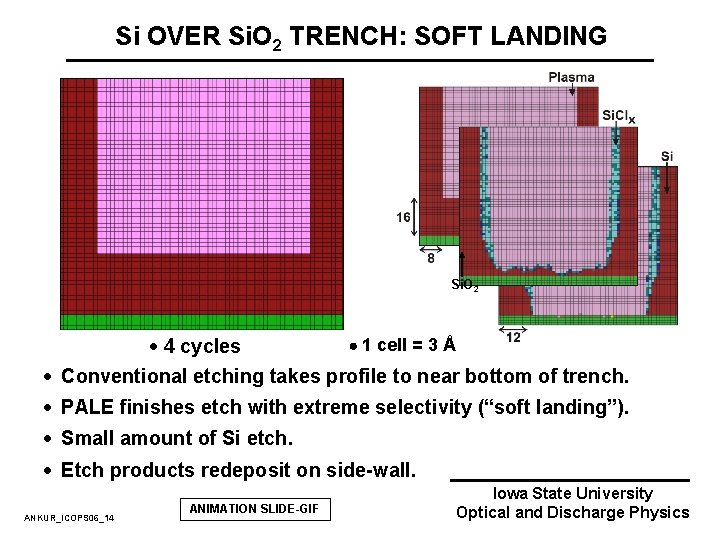
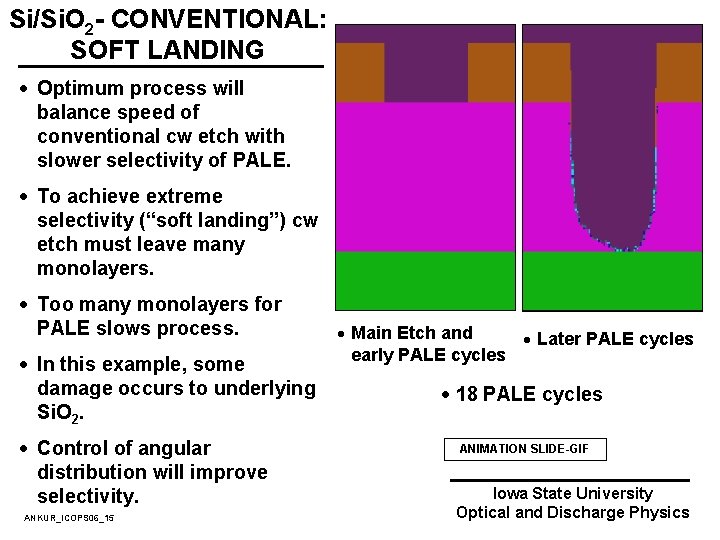
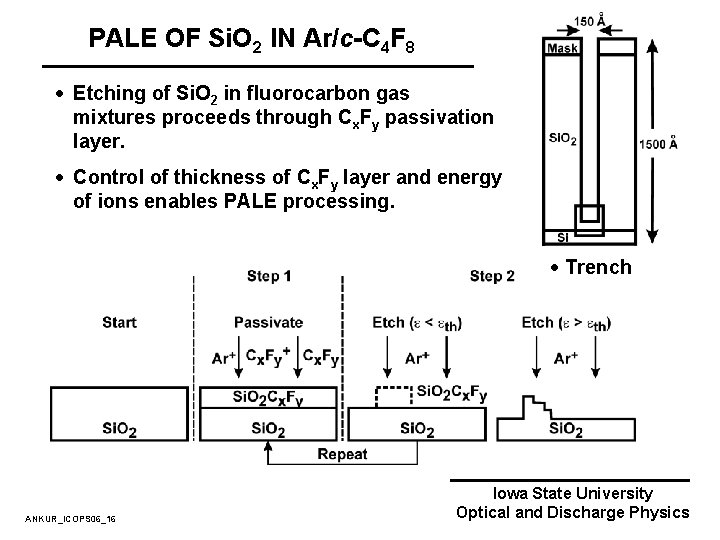
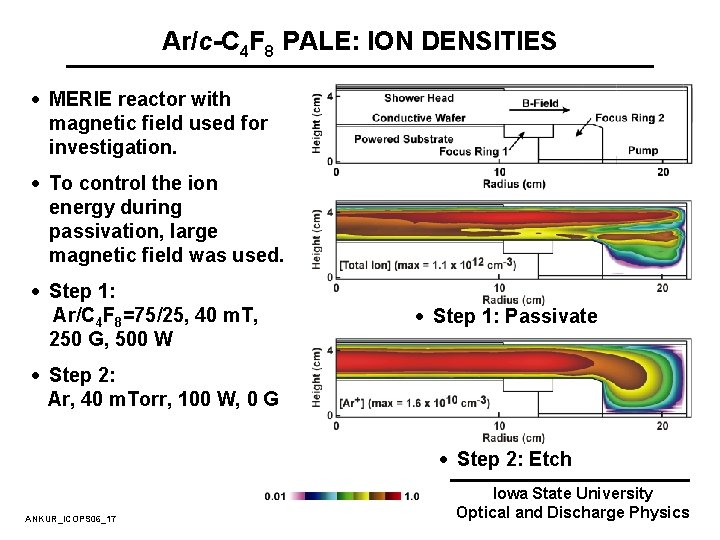
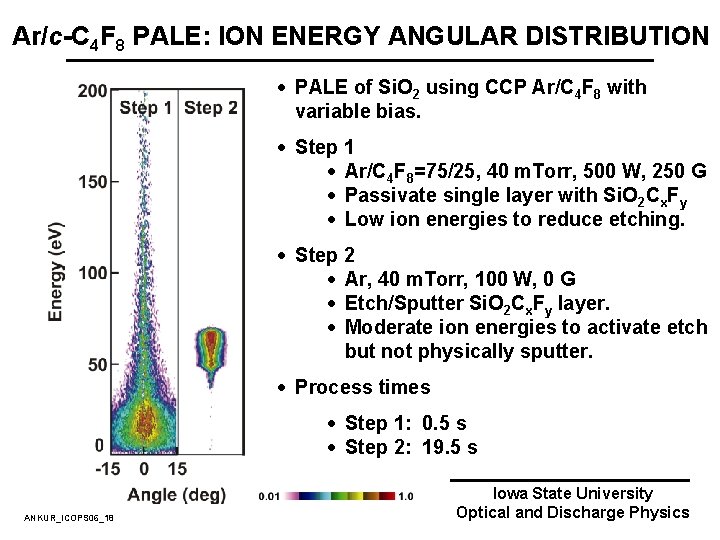
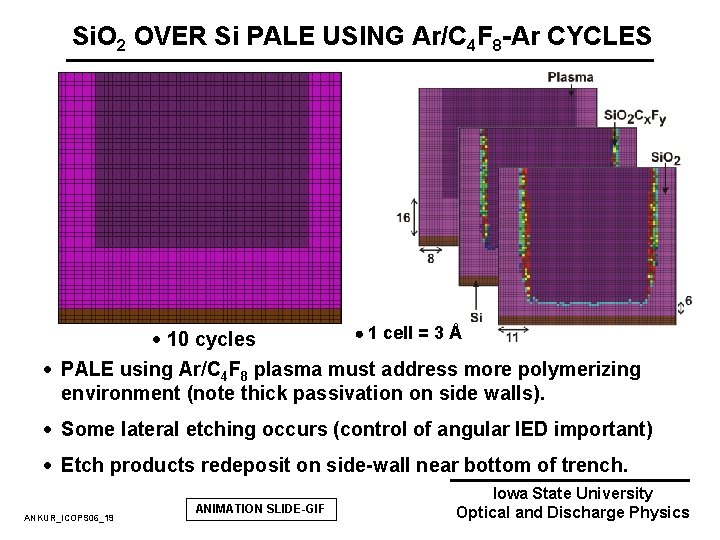
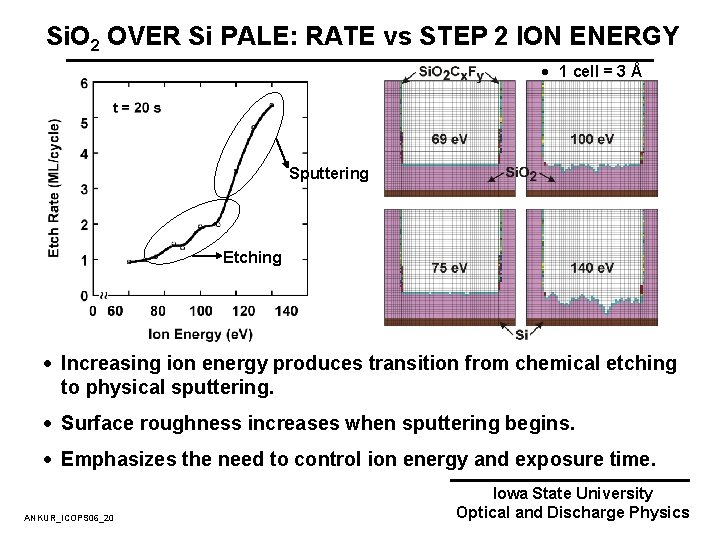
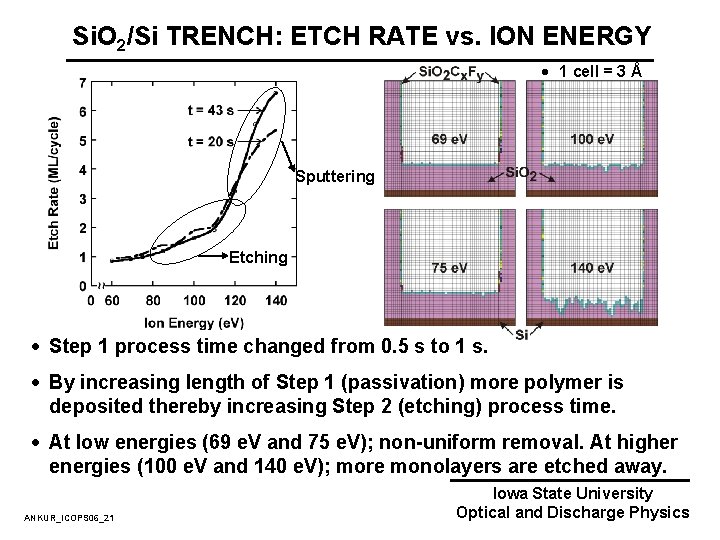
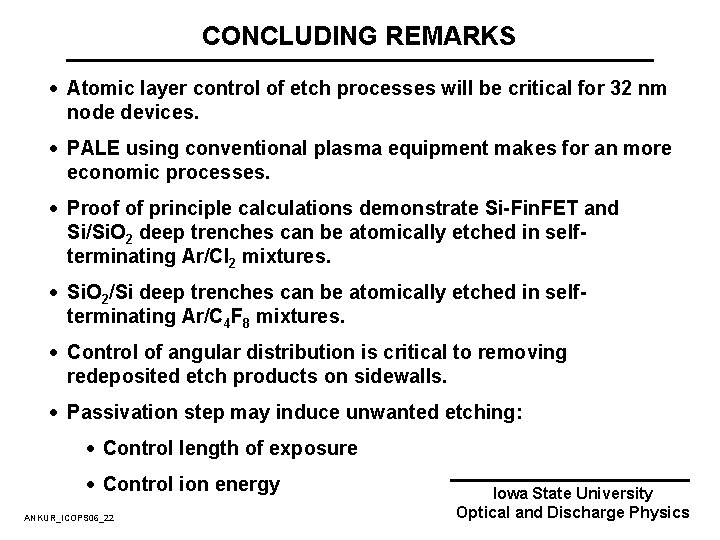
- Slides: 24
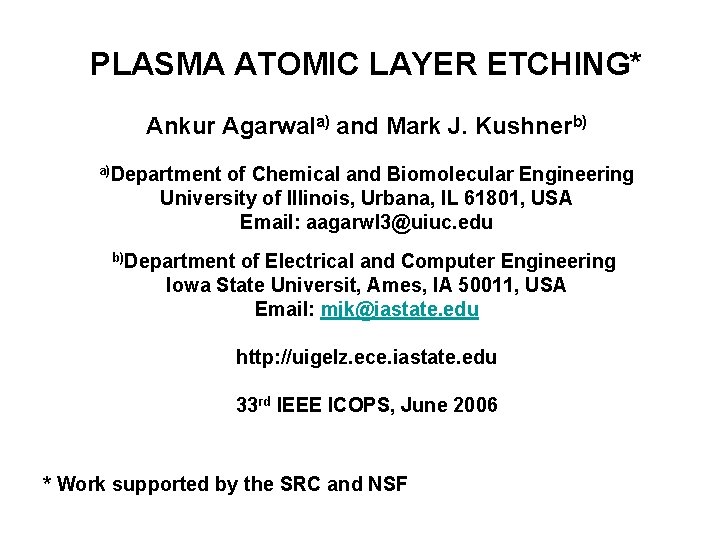
PLASMA ATOMIC LAYER ETCHING* Ankur Agarwala) and Mark J. Kushnerb) a)Department of Chemical and Biomolecular Engineering University of Illinois, Urbana, IL 61801, USA Email: aagarwl 3@uiuc. edu b)Department of Electrical and Computer Engineering Iowa State Universit, Ames, IA 50011, USA Email: mjk@iastate. edu http: //uigelz. ece. iastate. edu 33 rd IEEE ICOPS, June 2006 * Work supported by the SRC and NSF
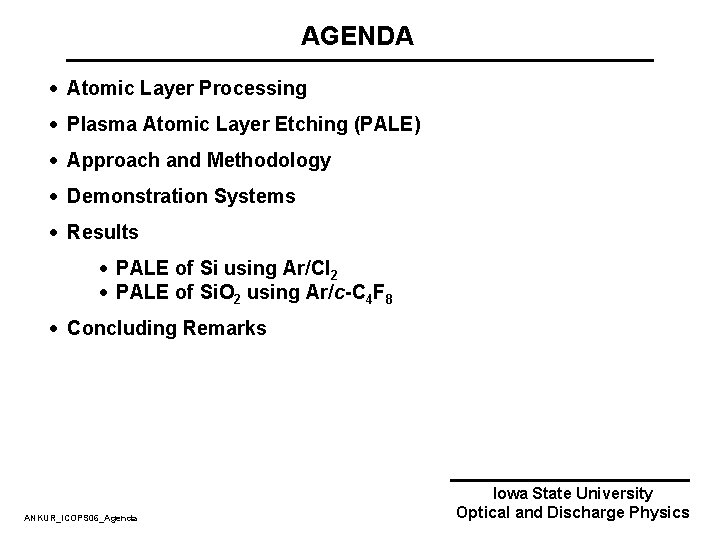
AGENDA · Atomic Layer Processing · Plasma Atomic Layer Etching (PALE) · Approach and Methodology · Demonstration Systems · Results · PALE of Si using Ar/Cl 2 · PALE of Si. O 2 using Ar/c-C 4 F 8 · Concluding Remarks ANKUR_ICOPS 06_Agenda Iowa State University Optical and Discharge Physics
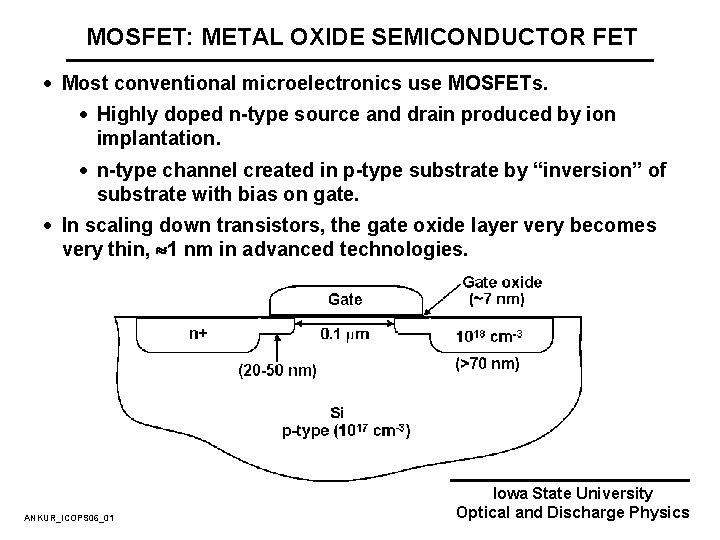
MOSFET: METAL OXIDE SEMICONDUCTOR FET · Most conventional microelectronics use MOSFETs. · Highly doped n-type source and drain produced by ion implantation. · n-type channel created in p-type substrate by “inversion” of substrate with bias on gate. · In scaling down transistors, the gate oxide layer very becomes very thin, 1 nm in advanced technologies. ANKUR_ICOPS 06_01 Iowa State University Optical and Discharge Physics
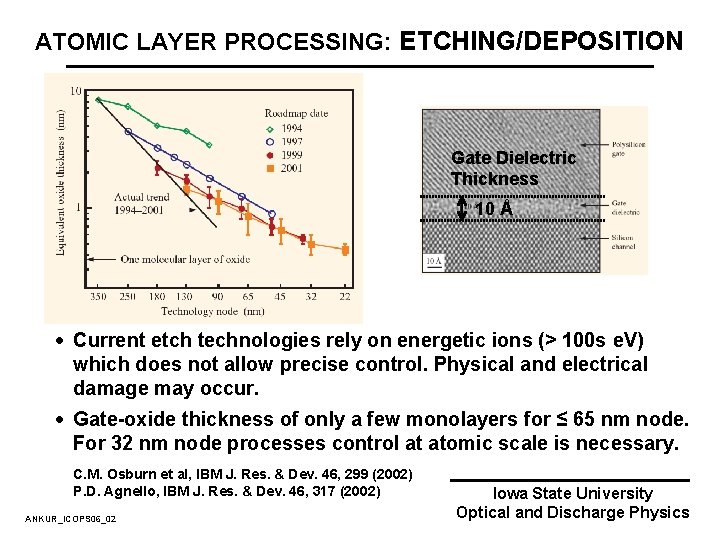
ATOMIC LAYER PROCESSING: ETCHING/DEPOSITION Gate Dielectric Thickness 10 Å · Current etch technologies rely on energetic ions (> 100 s e. V) which does not allow precise control. Physical and electrical damage may occur. · Gate-oxide thickness of only a few monolayers for ≤ 65 nm node. For 32 nm node processes control at atomic scale is necessary. C. M. Osburn et al, IBM J. Res. & Dev. 46, 299 (2002) P. D. Agnello, IBM J. Res. & Dev. 46, 317 (2002) ANKUR_ICOPS 06_02 Iowa State University Optical and Discharge Physics
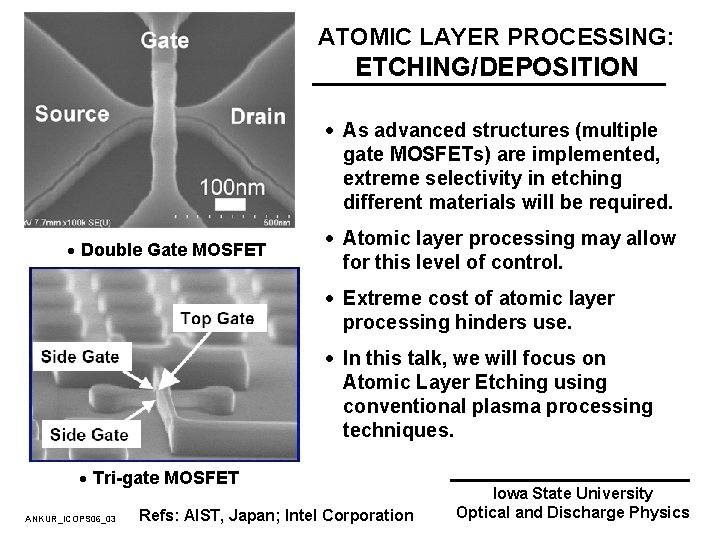
ATOMIC LAYER PROCESSING: ETCHING/DEPOSITION · As advanced structures (multiple gate MOSFETs) are implemented, extreme selectivity in etching different materials will be required. · Double Gate MOSFET · Atomic layer processing may allow for this level of control. · Extreme cost of atomic layer processing hinders use. · In this talk, we will focus on Atomic Layer Etching using conventional plasma processing techniques. · Tri-gate MOSFET ANKUR_ICOPS 06_03 Refs: AIST, Japan; Intel Corporation Iowa State University Optical and Discharge Physics
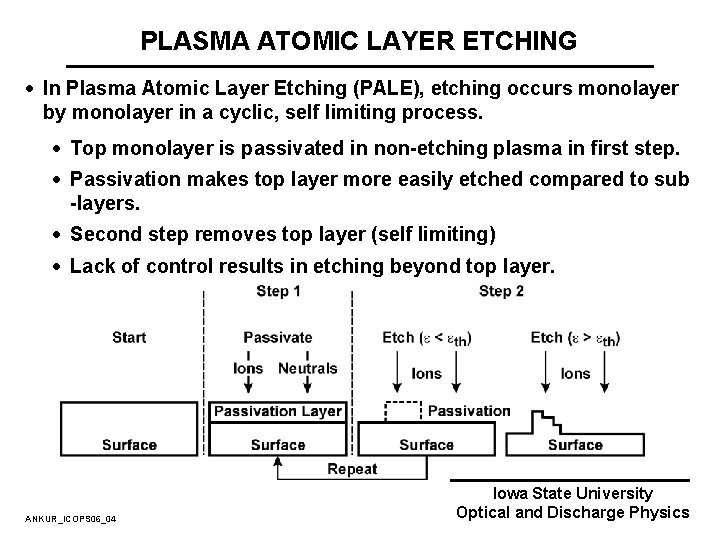
PLASMA ATOMIC LAYER ETCHING · In Plasma Atomic Layer Etching (PALE), etching occurs monolayer by monolayer in a cyclic, self limiting process. · Top monolayer is passivated in non-etching plasma in first step. · Passivation makes top layer more easily etched compared to sub -layers. · Second step removes top layer (self limiting) · Lack of control results in etching beyond top layer. ANKUR_ICOPS 06_04 Iowa State University Optical and Discharge Physics
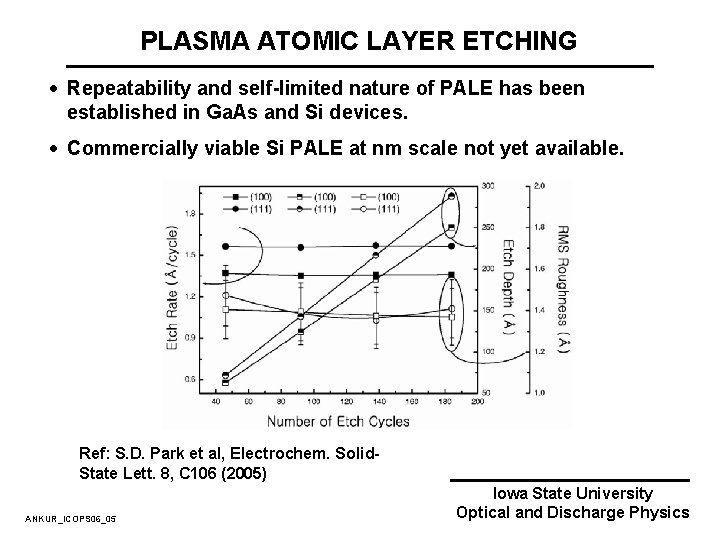
PLASMA ATOMIC LAYER ETCHING · Repeatability and self-limited nature of PALE has been established in Ga. As and Si devices. · Commercially viable Si PALE at nm scale not yet available. Ref: S. D. Park et al, Electrochem. Solid. State Lett. 8, C 106 (2005) ANKUR_ICOPS 06_05 Iowa State University Optical and Discharge Physics
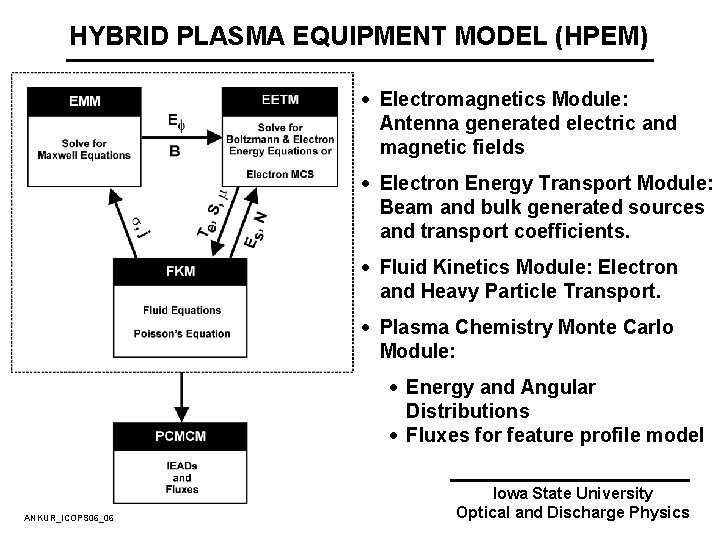
HYBRID PLASMA EQUIPMENT MODEL (HPEM) · Electromagnetics Module: Antenna generated electric and magnetic fields · Electron Energy Transport Module: Beam and bulk generated sources and transport coefficients. · Fluid Kinetics Module: Electron and Heavy Particle Transport. · Plasma Chemistry Monte Carlo Module: · Energy and Angular Distributions · Fluxes for feature profile model ANKUR_ICOPS 06_06 Iowa State University Optical and Discharge Physics
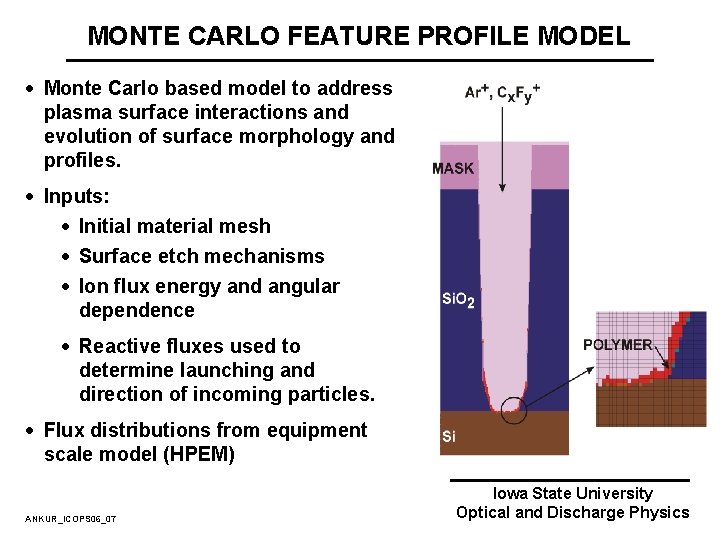
MONTE CARLO FEATURE PROFILE MODEL · Monte Carlo based model to address plasma surface interactions and evolution of surface morphology and profiles. · Inputs: · Initial material mesh · Surface etch mechanisms · Ion flux energy and angular dependence · Reactive fluxes used to determine launching and direction of incoming particles. · Flux distributions from equipment scale model (HPEM) ANKUR_ICOPS 06_07 Iowa State University Optical and Discharge Physics
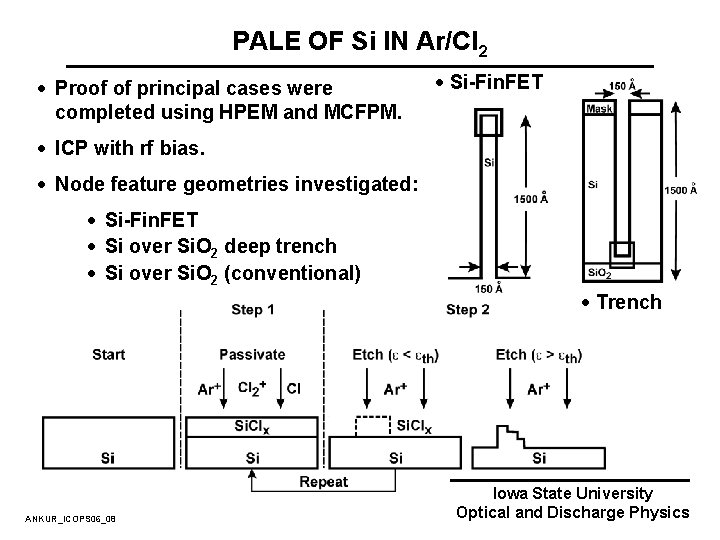
PALE OF Si IN Ar/Cl 2 · Proof of principal cases were completed using HPEM and MCFPM. · Si-Fin. FET · ICP with rf bias. · Node feature geometries investigated: · Si-Fin. FET · Si over Si. O 2 deep trench · Si over Si. O 2 (conventional) · Trench ANKUR_ICOPS 06_08 Iowa State University Optical and Discharge Physics
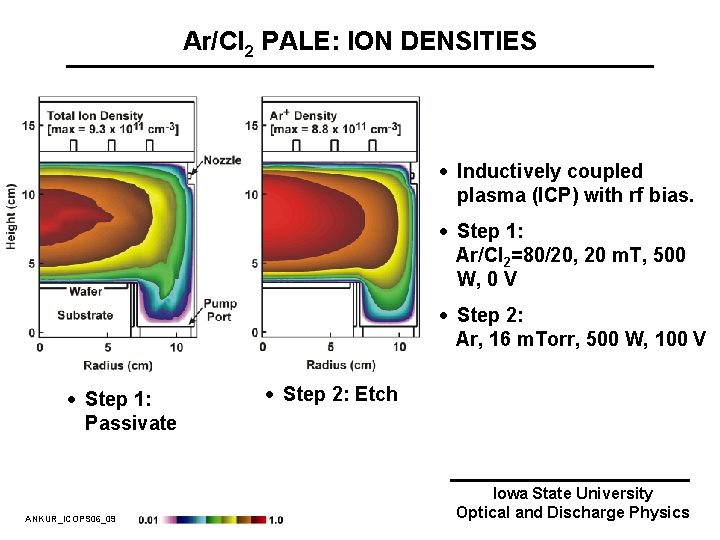
Ar/Cl 2 PALE: ION DENSITIES · Inductively coupled plasma (ICP) with rf bias. · Step 1: Ar/Cl 2=80/20, 20 m. T, 500 W, 0 V · Step 2: Ar, 16 m. Torr, 500 W, 100 V · Step 1: Passivate ANKUR_ICOPS 06_09 · Step 2: Etch Iowa State University Optical and Discharge Physics
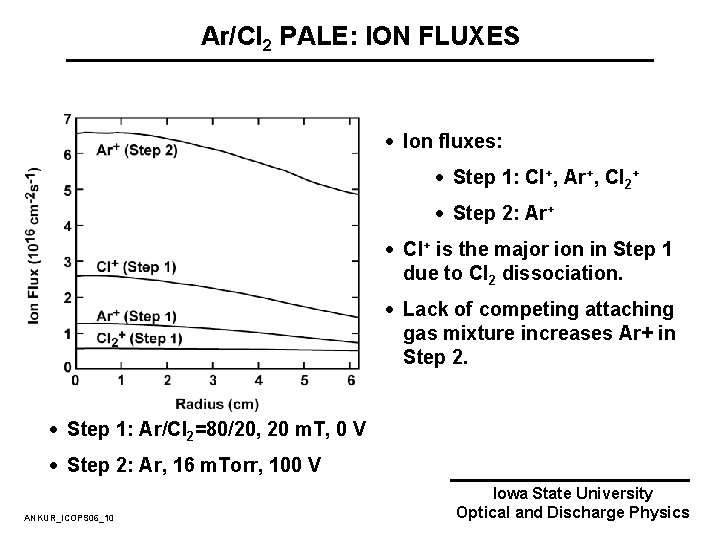
Ar/Cl 2 PALE: ION FLUXES · Ion fluxes: · Step 1: Cl+, Ar+, Cl 2+ · Step 2: Ar+ · Cl+ is the major ion in Step 1 due to Cl 2 dissociation. · Lack of competing attaching gas mixture increases Ar+ in Step 2. · Step 1: Ar/Cl 2=80/20, 20 m. T, 0 V · Step 2: Ar, 16 m. Torr, 100 V ANKUR_ICOPS 06_10 Iowa State University Optical and Discharge Physics
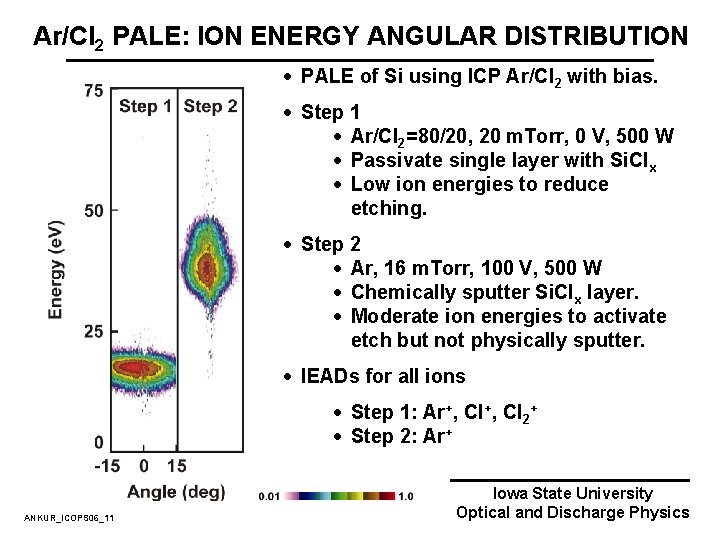
Ar/Cl 2 PALE: ION ENERGY ANGULAR DISTRIBUTION · PALE of Si using ICP Ar/Cl 2 with bias. · Step 1 · Ar/Cl 2=80/20, 20 m. Torr, 0 V, 500 W · Passivate single layer with Si. Clx · Low ion energies to reduce etching. · Step 2 · Ar, 16 m. Torr, 100 V, 500 W · Chemically sputter Si. Clx layer. · Moderate ion energies to activate etch but not physically sputter. · IEADs for all ions · Step 1: Ar+, Cl 2+ · Step 2: Ar+ ANKUR_ICOPS 06_11 Iowa State University Optical and Discharge Physics
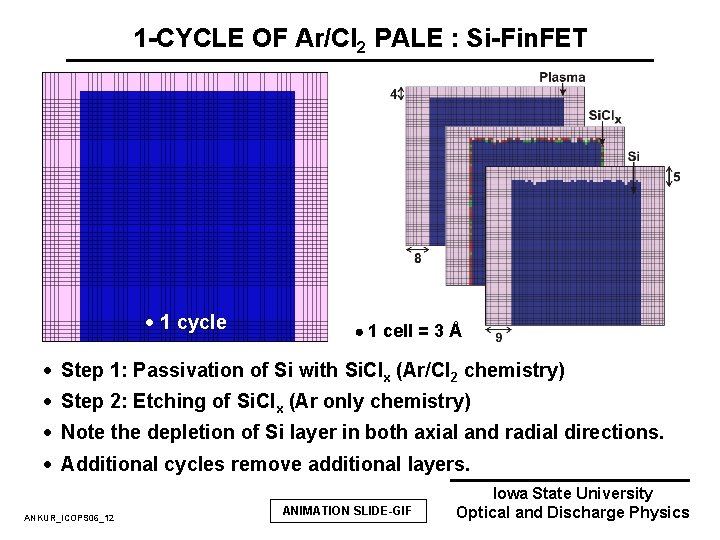
1 -CYCLE OF Ar/Cl 2 PALE : Si-Fin. FET · 1 cycle 1 cell = 3 Å · Step 1: Passivation of Si with Si. Clx (Ar/Cl 2 chemistry) · Step 2: Etching of Si. Clx (Ar only chemistry) · Note the depletion of Si layer in both axial and radial directions. · Additional cycles remove additional layers. ANKUR_ICOPS 06_12 ANIMATION SLIDE-GIF Iowa State University Optical and Discharge Physics
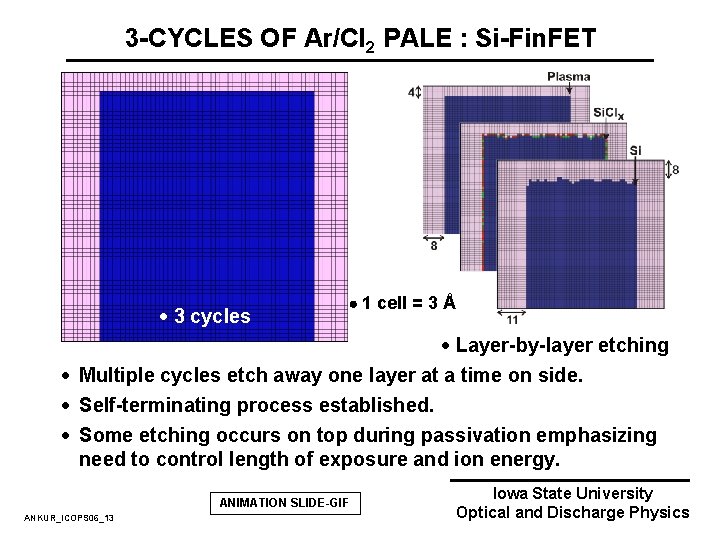
3 -CYCLES OF Ar/Cl 2 PALE : Si-Fin. FET · 3 cycles 1 cell = 3 Å · Layer-by-layer etching · Multiple cycles etch away one layer at a time on side. · Self-terminating process established. · Some etching occurs on top during passivation emphasizing need to control length of exposure and ion energy. ANIMATION SLIDE-GIF ANKUR_ICOPS 06_13 Iowa State University Optical and Discharge Physics
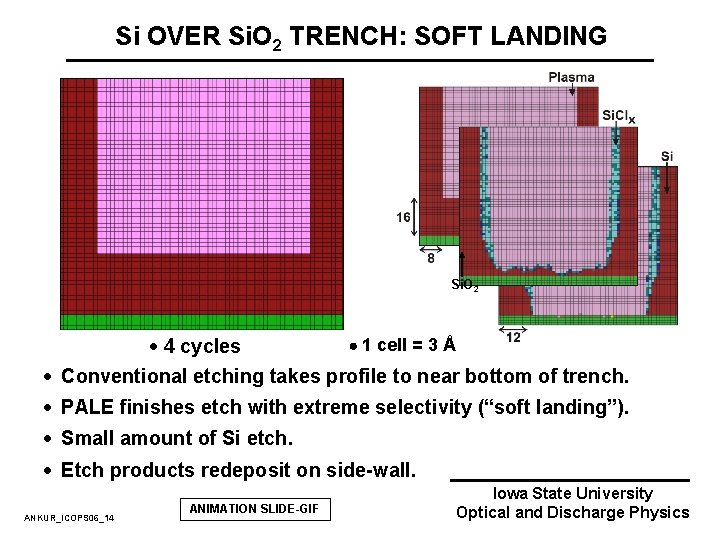
Si OVER Si. O 2 TRENCH: SOFT LANDING Si. O 2 1 cell = 3 Å · 4 cycles · Conventional etching takes profile to near bottom of trench. · PALE finishes etch with extreme selectivity (“soft landing”). · Small amount of Si etch. · Etch products redeposit on side-wall. ANKUR_ICOPS 06_14 ANIMATION SLIDE-GIF Iowa State University Optical and Discharge Physics
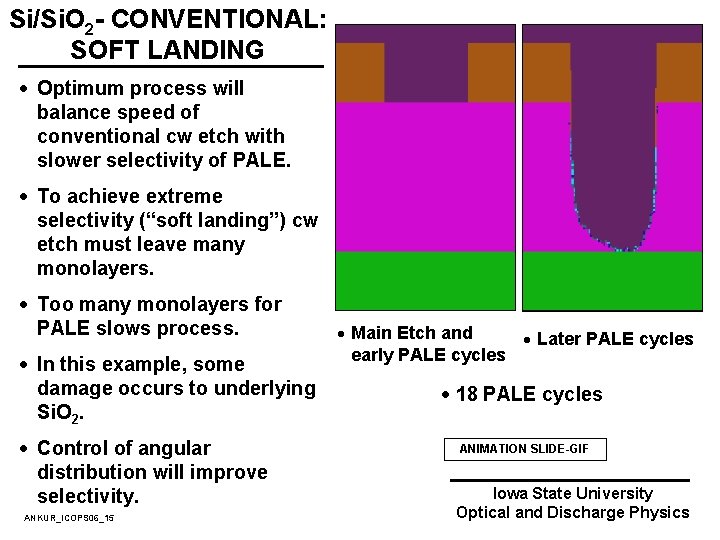
Si/Si. O 2 - CONVENTIONAL: SOFT LANDING · Optimum process will balance speed of conventional cw etch with slower selectivity of PALE. · To achieve extreme selectivity (“soft landing”) cw etch must leave many monolayers. · Too many monolayers for PALE slows process. · In this example, some damage occurs to underlying Si. O 2. · Control of angular distribution will improve selectivity. ANKUR_ICOPS 06_15 · Main Etch and · Later PALE cycles early PALE cycles · 18 PALE cycles ANIMATION SLIDE-GIF Iowa State University Optical and Discharge Physics
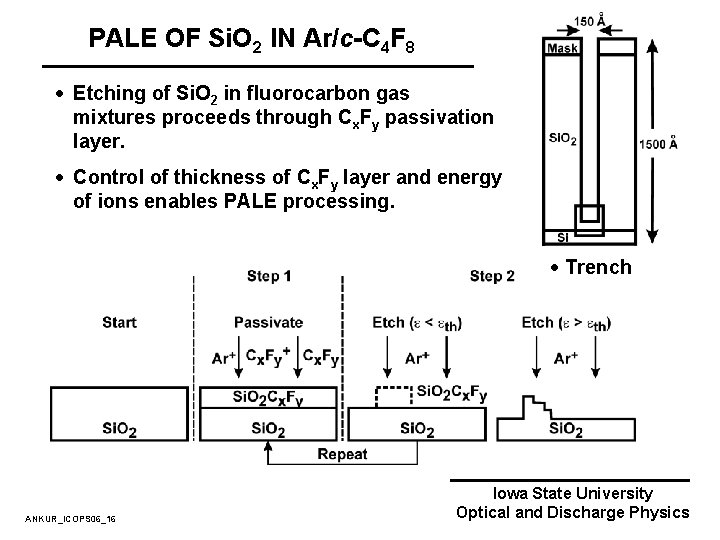
PALE OF Si. O 2 IN Ar/c-C 4 F 8 · Etching of Si. O 2 in fluorocarbon gas mixtures proceeds through Cx. Fy passivation layer. · Control of thickness of Cx. Fy layer and energy of ions enables PALE processing. · Trench ANKUR_ICOPS 06_16 Iowa State University Optical and Discharge Physics
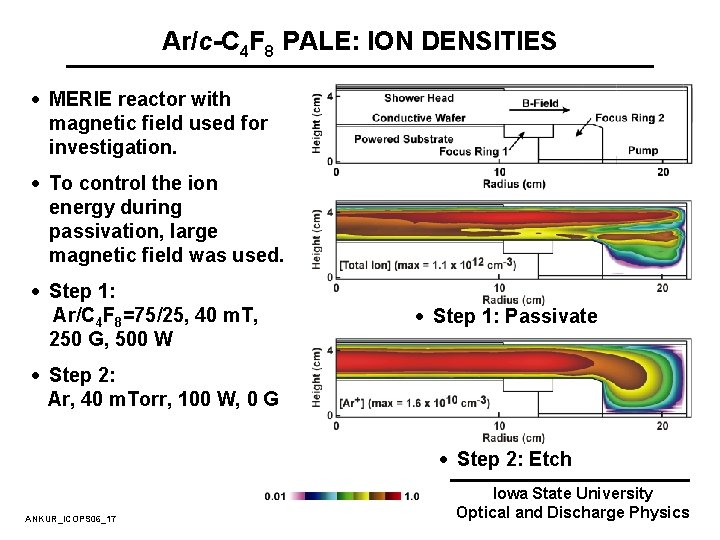
Ar/c-C 4 F 8 PALE: ION DENSITIES · MERIE reactor with magnetic field used for investigation. · To control the ion energy during passivation, large magnetic field was used. · Step 1: Ar/C 4 F 8=75/25, 40 m. T, 250 G, 500 W · Step 1: Passivate · Step 2: Ar, 40 m. Torr, 100 W, 0 G · Step 2: Etch ANKUR_ICOPS 06_17 Iowa State University Optical and Discharge Physics
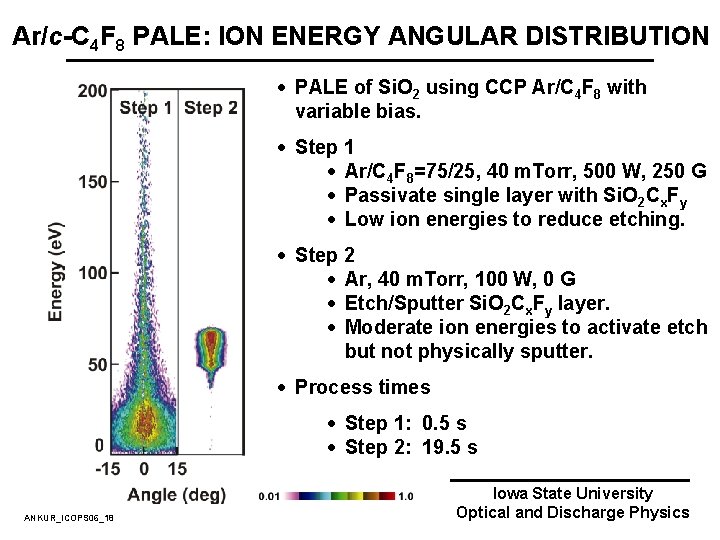
Ar/c-C 4 F 8 PALE: ION ENERGY ANGULAR DISTRIBUTION · PALE of Si. O 2 using CCP Ar/C 4 F 8 with variable bias. · Step 1 · Ar/C 4 F 8=75/25, 40 m. Torr, 500 W, 250 G · Passivate single layer with Si. O 2 Cx. Fy · Low ion energies to reduce etching. · Step 2 · Ar, 40 m. Torr, 100 W, 0 G · Etch/Sputter Si. O 2 Cx. Fy layer. · Moderate ion energies to activate etch but not physically sputter. · Process times · Step 1: 0. 5 s · Step 2: 19. 5 s ANKUR_ICOPS 06_18 Iowa State University Optical and Discharge Physics
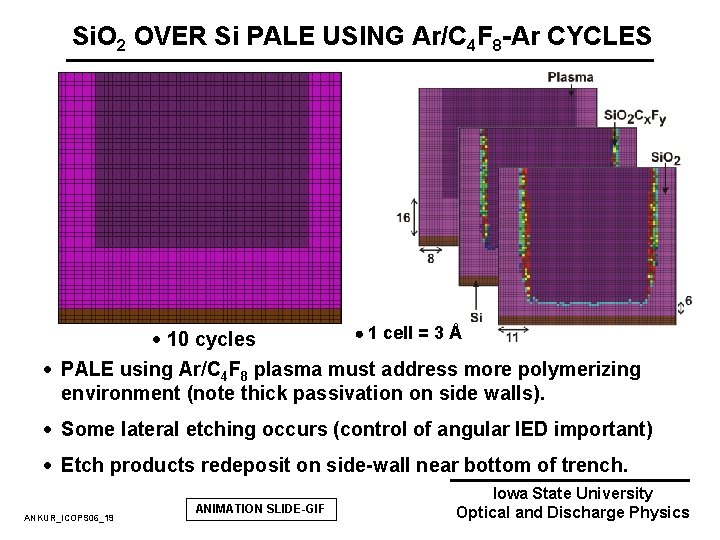
Si. O 2 OVER Si PALE USING Ar/C 4 F 8 -Ar CYCLES 1 cell = 3 Å · 10 cycles · PALE using Ar/C 4 F 8 plasma must address more polymerizing environment (note thick passivation on side walls). · Some lateral etching occurs (control of angular IED important) · Etch products redeposit on side-wall near bottom of trench. ANKUR_ICOPS 06_19 ANIMATION SLIDE-GIF Iowa State University Optical and Discharge Physics
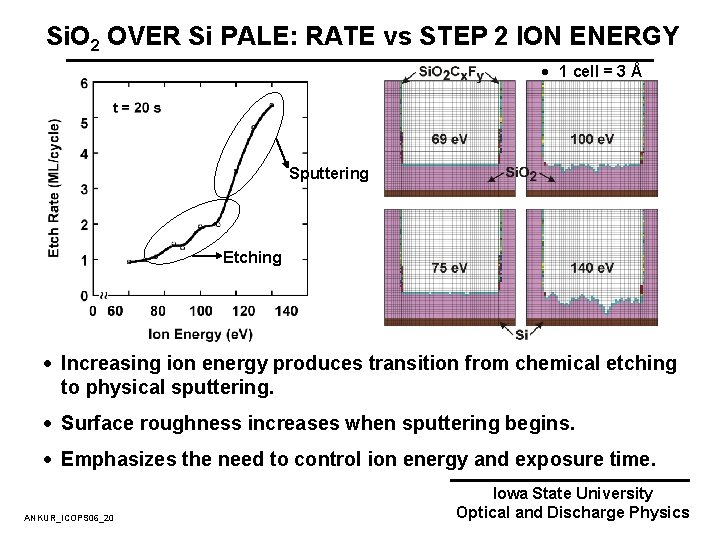
Si. O 2 OVER Si PALE: RATE vs STEP 2 ION ENERGY · 1 cell = 3 Å Sputtering Etching · Increasing ion energy produces transition from chemical etching to physical sputtering. · Surface roughness increases when sputtering begins. · Emphasizes the need to control ion energy and exposure time. ANKUR_ICOPS 06_20 Iowa State University Optical and Discharge Physics
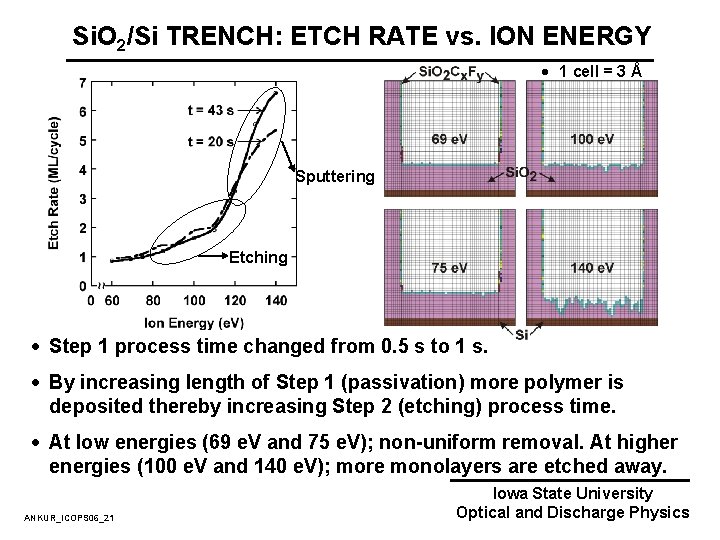
Si. O 2/Si TRENCH: ETCH RATE vs. ION ENERGY · 1 cell = 3 Å Sputtering Etching · Step 1 process time changed from 0. 5 s to 1 s. · By increasing length of Step 1 (passivation) more polymer is deposited thereby increasing Step 2 (etching) process time. · At low energies (69 e. V and 75 e. V); non-uniform removal. At higher energies (100 e. V and 140 e. V); more monolayers are etched away. ANKUR_ICOPS 06_21 Iowa State University Optical and Discharge Physics
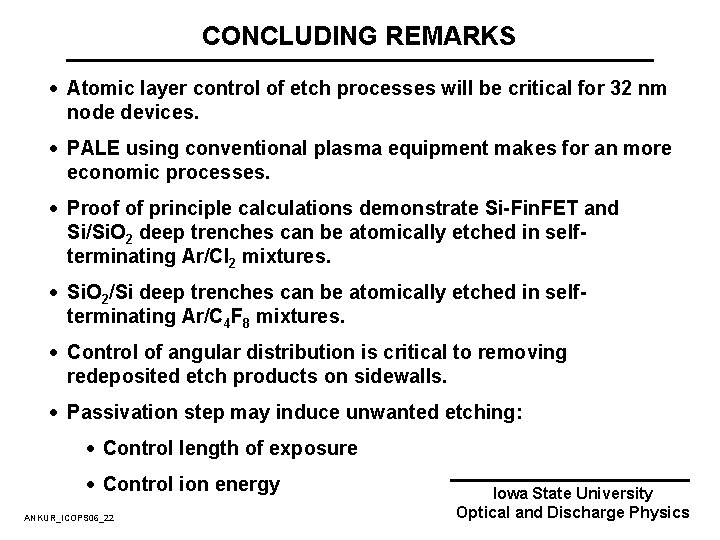
CONCLUDING REMARKS · Atomic layer control of etch processes will be critical for 32 nm node devices. · PALE using conventional plasma equipment makes for an more economic processes. · Proof of principle calculations demonstrate Si-Fin. FET and Si/Si. O 2 deep trenches can be atomically etched in selfterminating Ar/Cl 2 mixtures. · Si. O 2/Si deep trenches can be atomically etched in selfterminating Ar/C 4 F 8 mixtures. · Control of angular distribution is critical to removing redeposited etch products on sidewalls. · Passivation step may induce unwanted etching: · Control length of exposure · Control ion energy ANKUR_ICOPS 06_22 Iowa State University Optical and Discharge Physics
Atomic layer etching
Etch rate definition
Wet etching vs dry etching
Soonmin bae
Dr ankur sinha
Ankur taly
Relative formula mass of hcl
How to calculate abundance of isotopes
Differentiate between atomic number and mass number
Cervident ss white
Fig 19
Pharynx
Secure socket layer and transport layer security
Secure socket layer and transport layer security
Secure socket layer and transport layer security
Secure socket layer and transport layer security
Patterns of the periodic table
Atomic radius
Atomic number vs atomic radius
Atomic layer deposition for semiconductors
Atomic layer deposition wiki
Atomic layer deposition
Serial number restoration
High aspect ratio contact
Etch